Reaction kinetics 1 R 1 The rate of
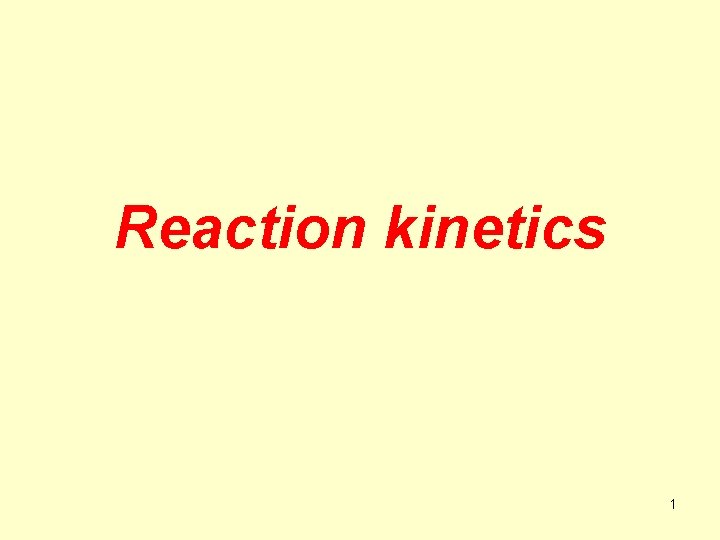
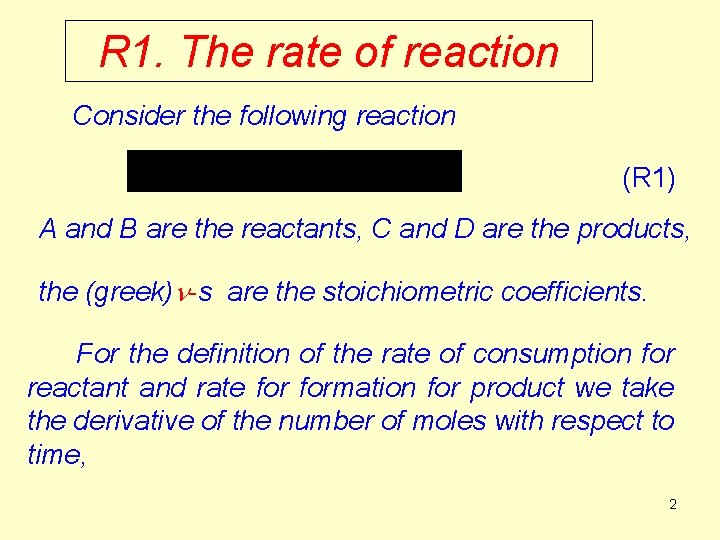
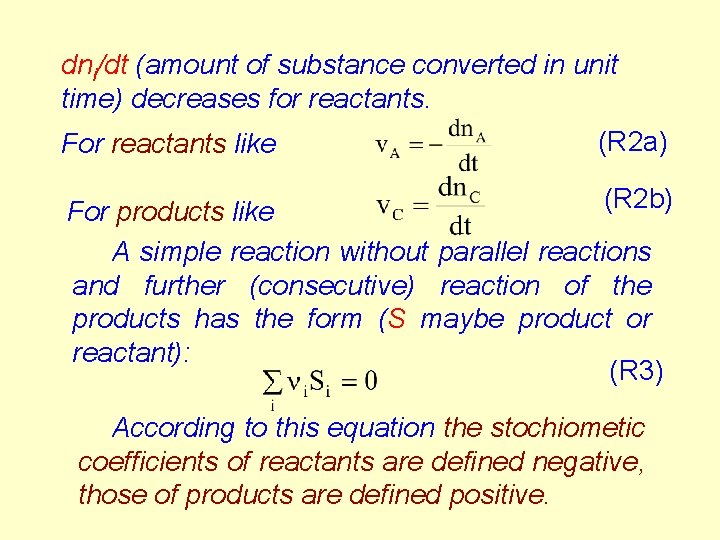
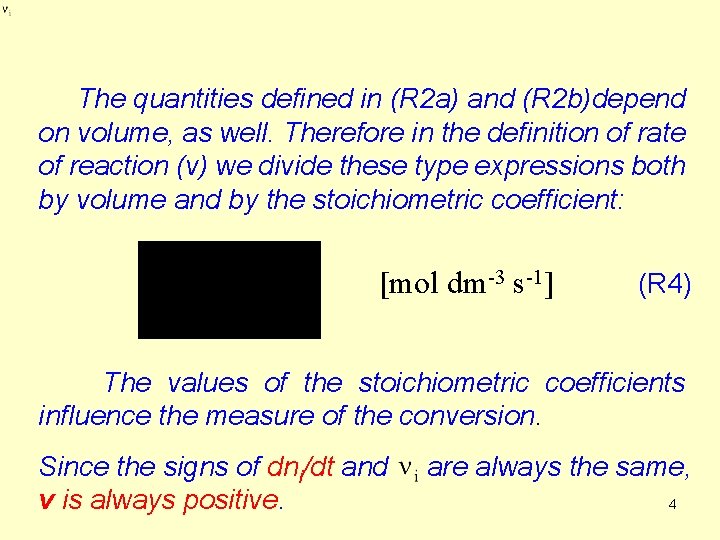
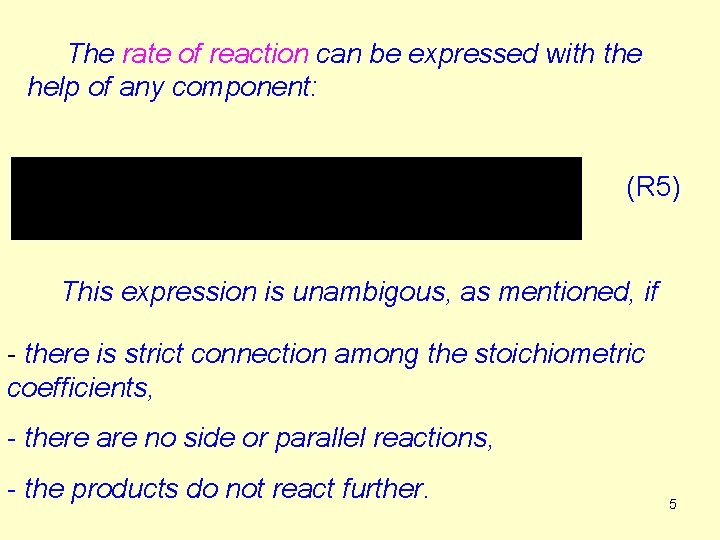
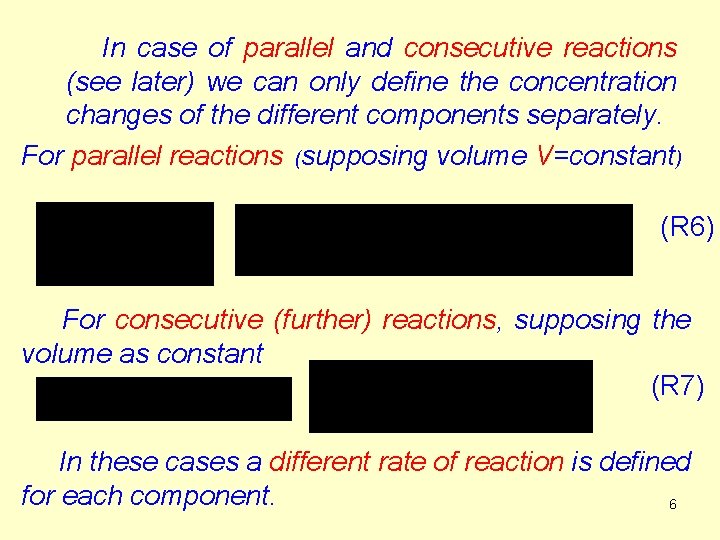
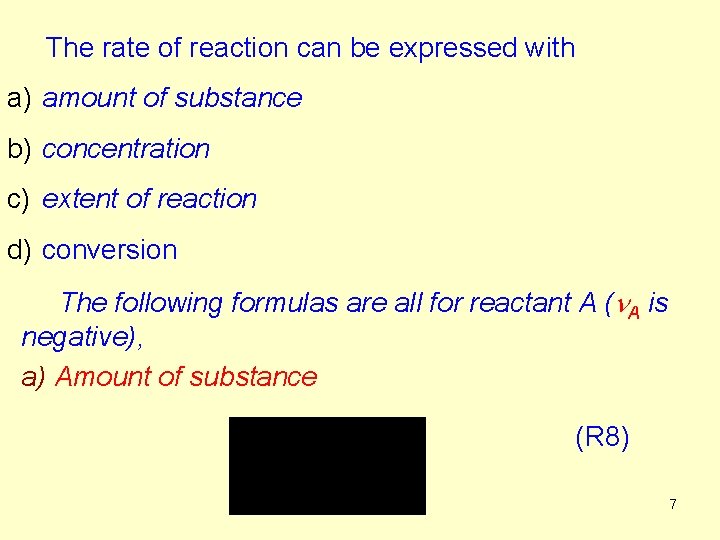
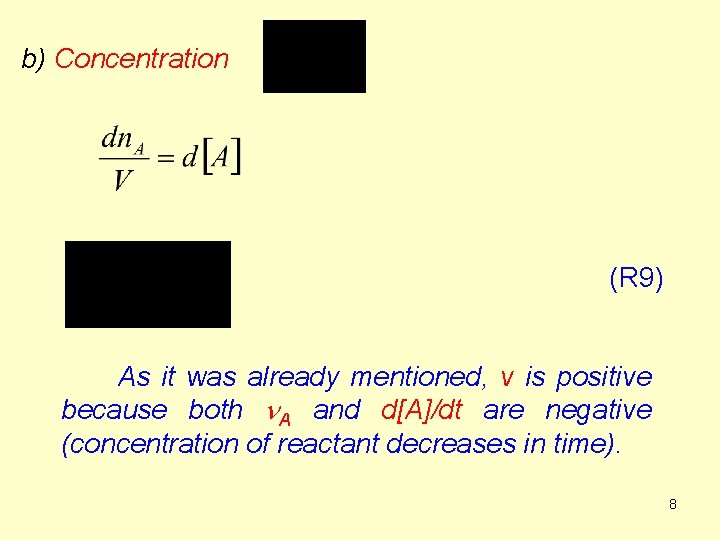
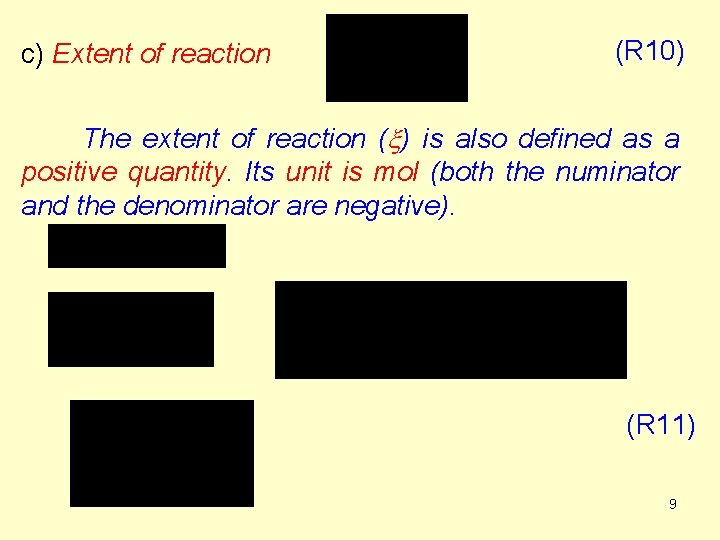
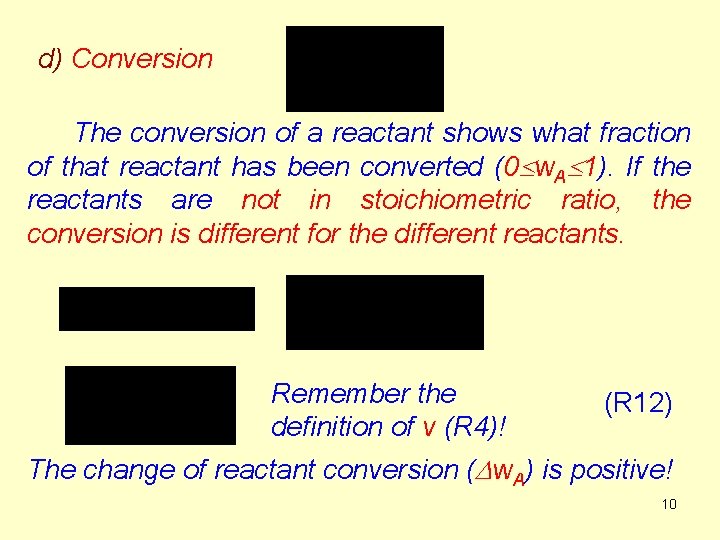
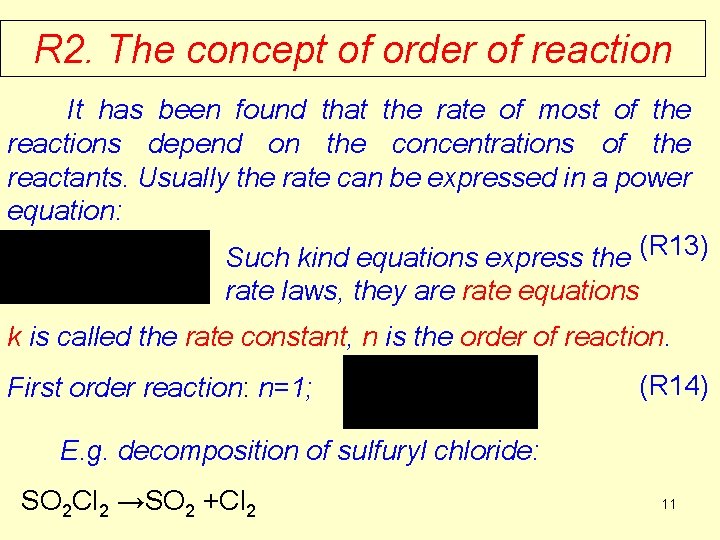
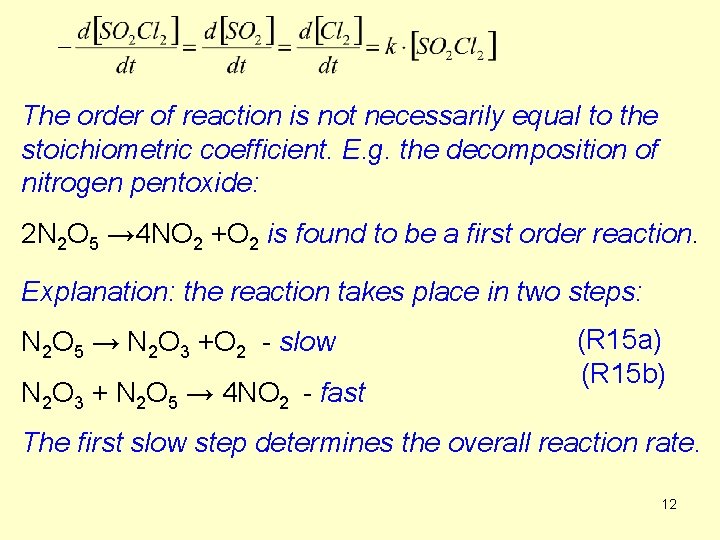
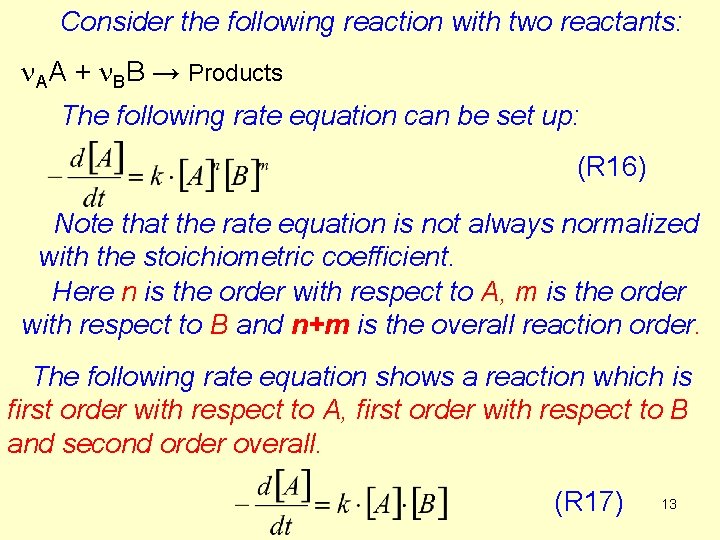
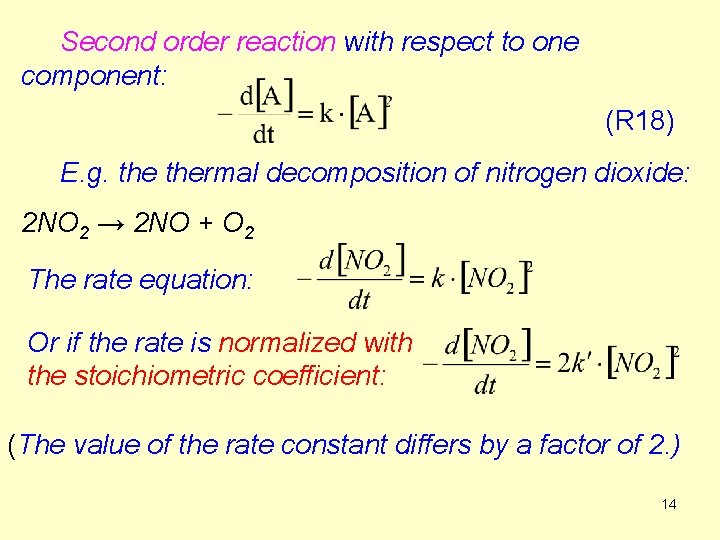
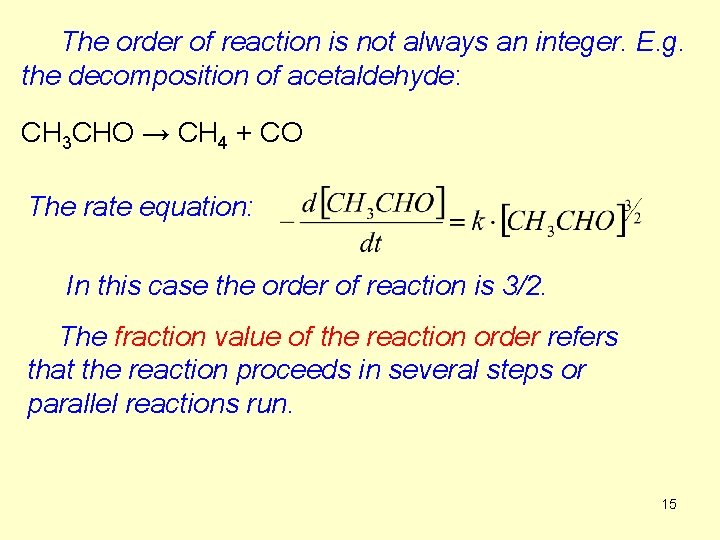
![The unit of rate constant depends on the order of reaction. First order [k] The unit of rate constant depends on the order of reaction. First order [k]](https://slidetodoc.com/presentation_image_h/9fcac2c20e98a062fd11eb0e061bde2e/image-16.jpg)
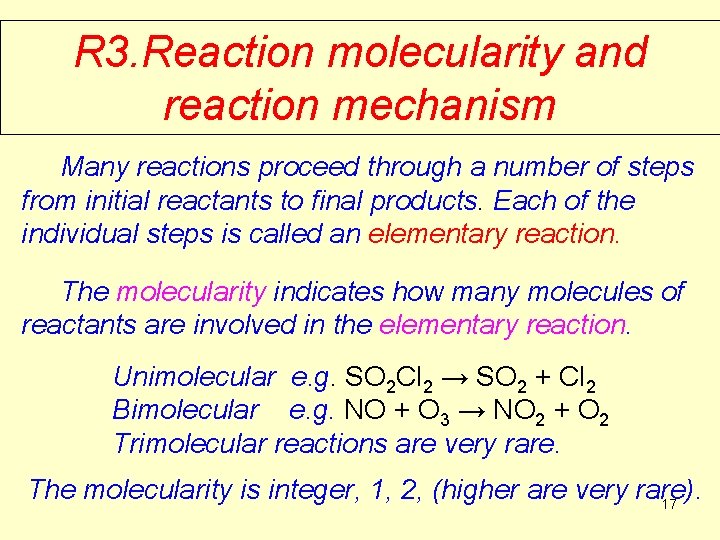
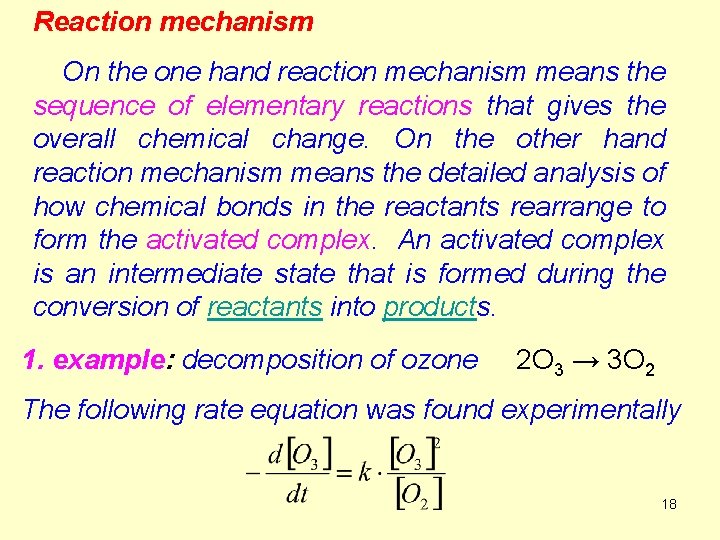
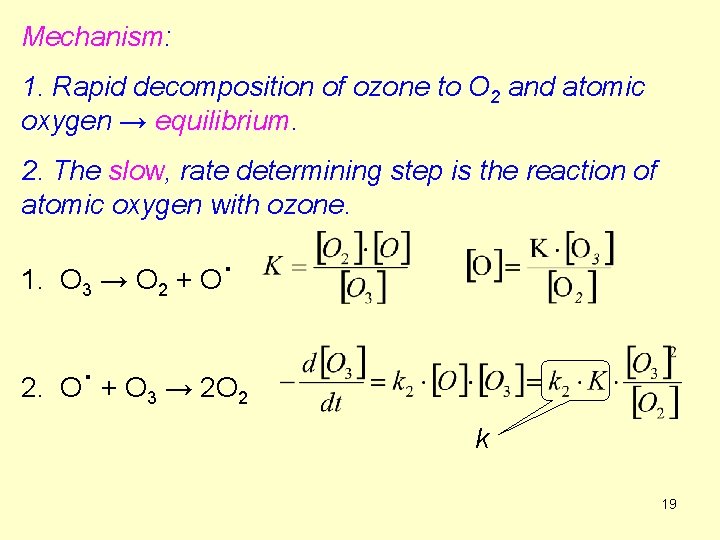
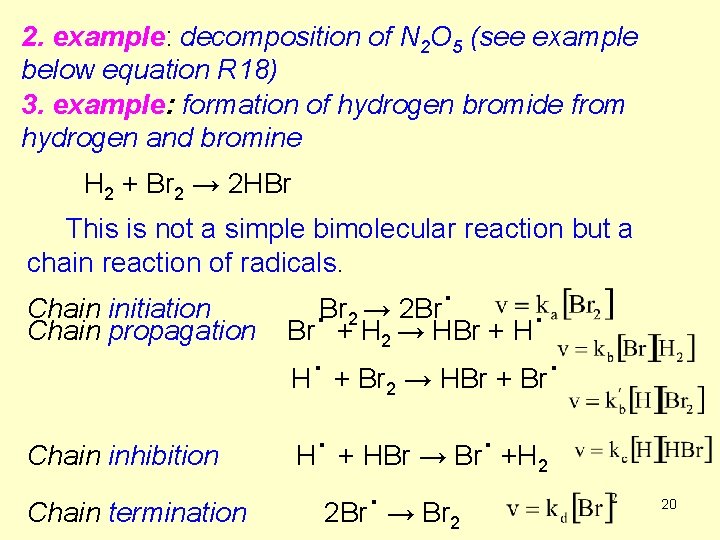
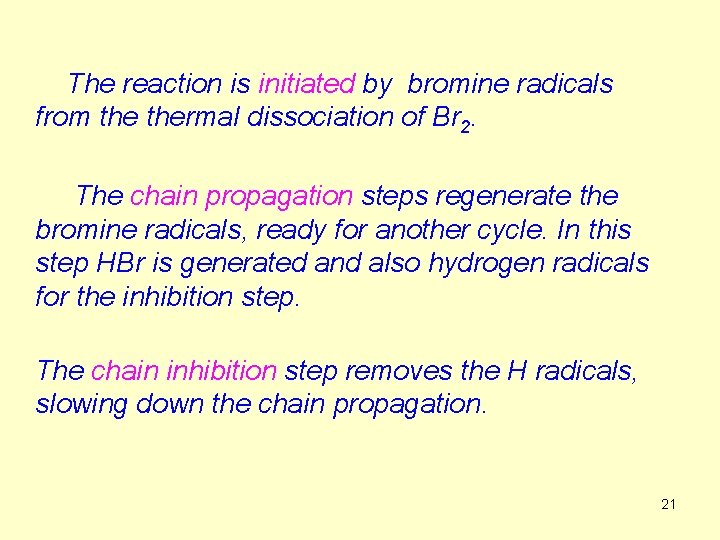
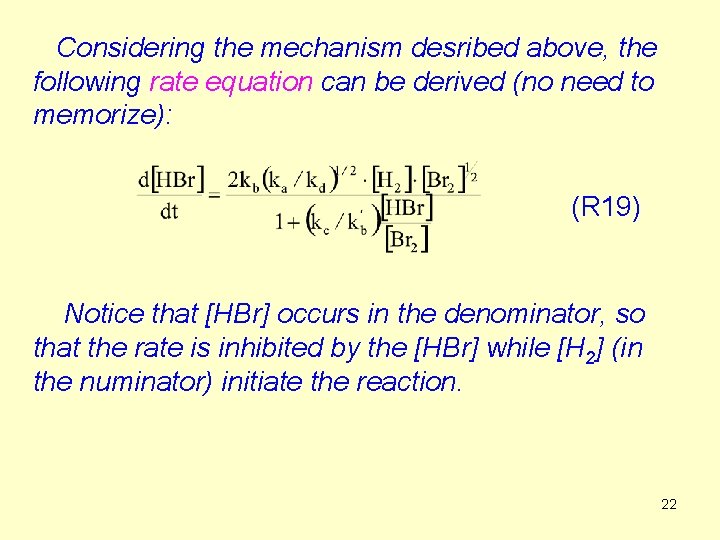
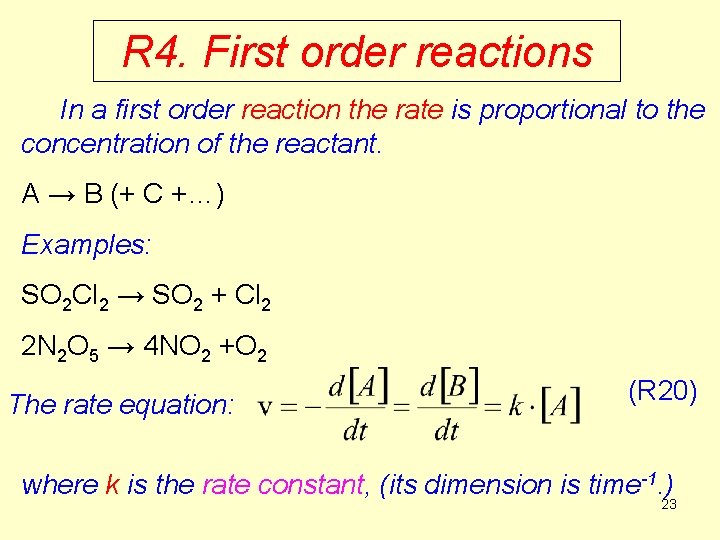
![Separate the variables and integrate from t = 0 (conc. = [A 0]) to Separate the variables and integrate from t = 0 (conc. = [A 0]) to](https://slidetodoc.com/presentation_image_h/9fcac2c20e98a062fd11eb0e061bde2e/image-24.jpg)
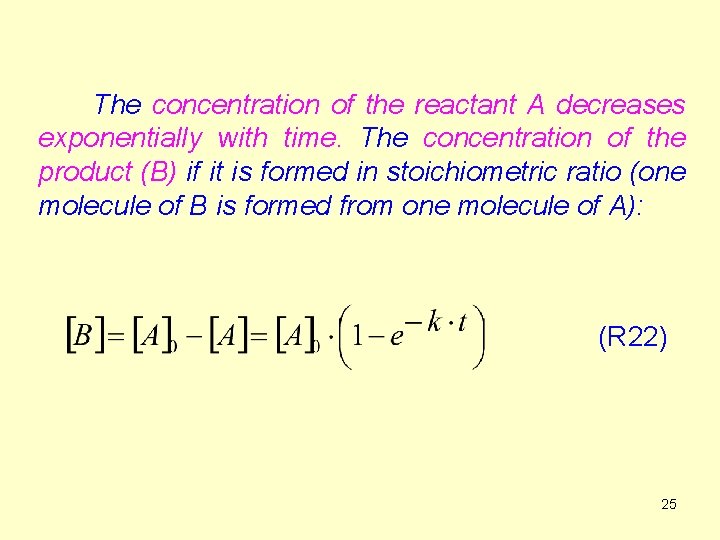
![The concentrations as functions of time. concentration [A]0 [B] [A] time 26 The concentrations as functions of time. concentration [A]0 [B] [A] time 26](https://slidetodoc.com/presentation_image_h/9fcac2c20e98a062fd11eb0e061bde2e/image-26.jpg)
![Linear plot (R 21 b) ln[A]0 experimental data a tga = -k time 27 Linear plot (R 21 b) ln[A]0 experimental data a tga = -k time 27](https://slidetodoc.com/presentation_image_h/9fcac2c20e98a062fd11eb0e061bde2e/image-27.jpg)
![If we plot ln[A] against time and the data points lie on a staight If we plot ln[A] against time and the data points lie on a staight](https://slidetodoc.com/presentation_image_h/9fcac2c20e98a062fd11eb0e061bde2e/image-28.jpg)
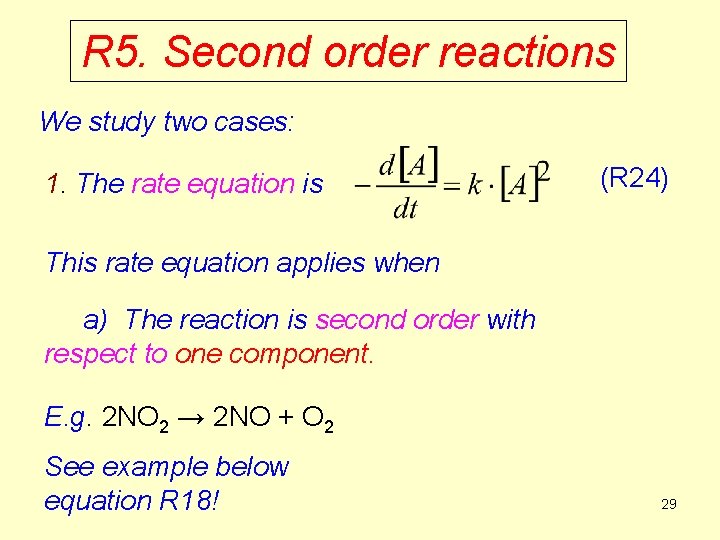
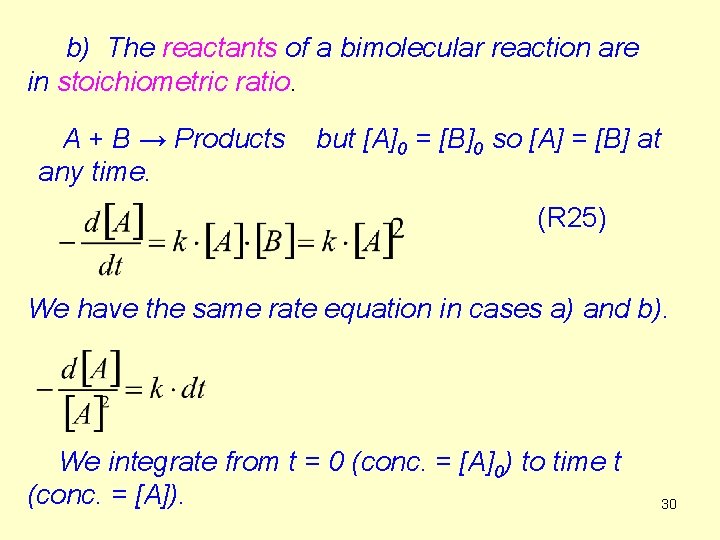
![(The integral of 1/[A]2 is -1/[A] +const. ) The integrated form of the rate (The integral of 1/[A]2 is -1/[A] +const. ) The integrated form of the rate](https://slidetodoc.com/presentation_image_h/9fcac2c20e98a062fd11eb0e061bde2e/image-31.jpg)
![Linear plot (R 26) 1/[A] experimental data a tga = k 1/[A]0 time 32 Linear plot (R 26) 1/[A] experimental data a tga = k 1/[A]0 time 32](https://slidetodoc.com/presentation_image_h/9fcac2c20e98a062fd11eb0e061bde2e/image-32.jpg)
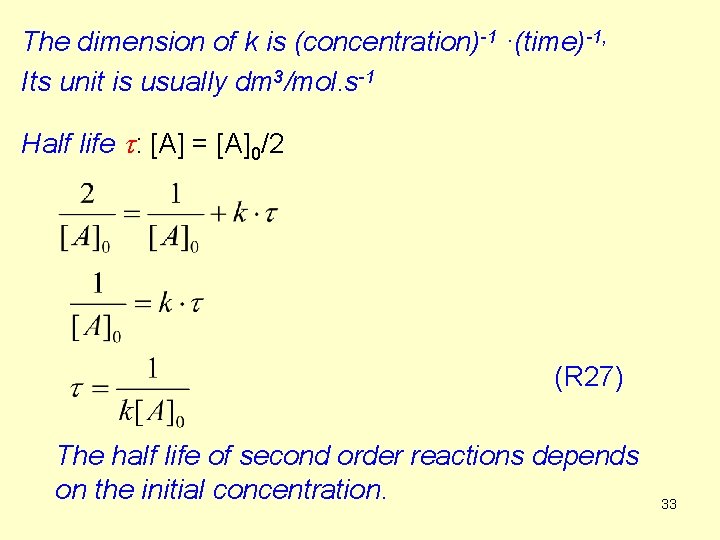
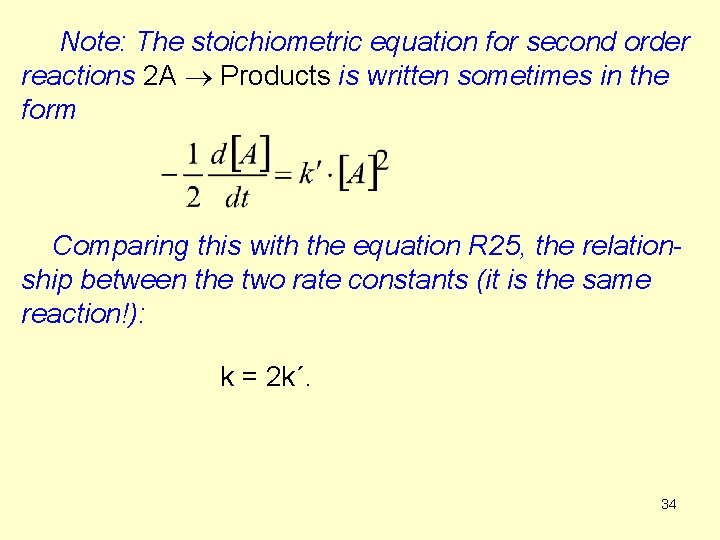
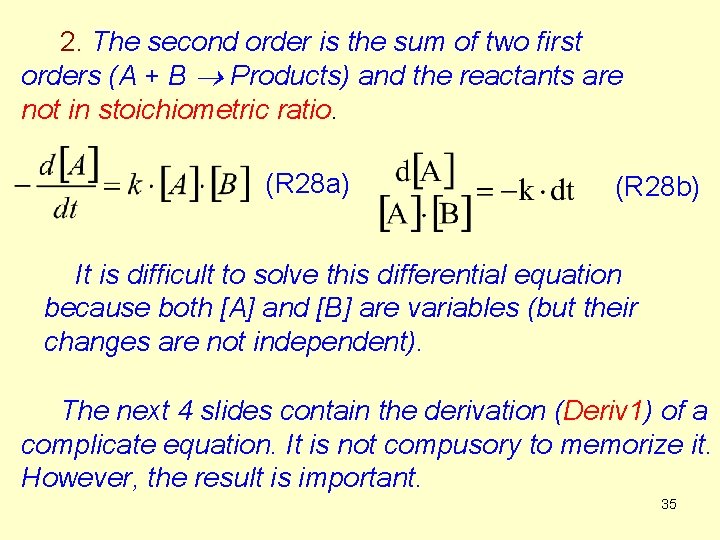
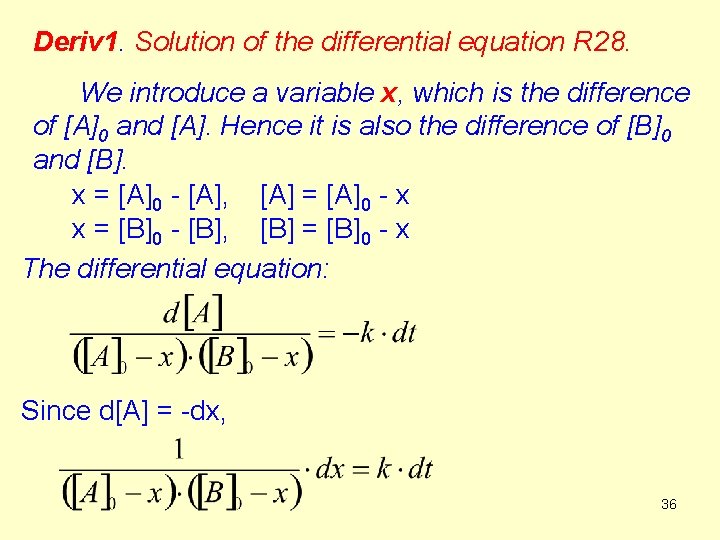
![To alter the left hand side consider the following identity: If we substitute [A]0 To alter the left hand side consider the following identity: If we substitute [A]0](https://slidetodoc.com/presentation_image_h/9fcac2c20e98a062fd11eb0e061bde2e/image-37.jpg)
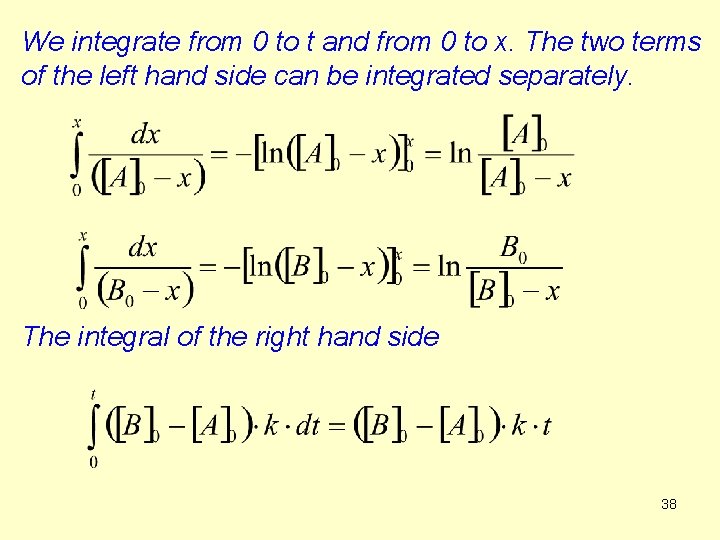
![So the solution of the differential equation Rearrange this equation considering that [A]0 -x So the solution of the differential equation Rearrange this equation considering that [A]0 -x](https://slidetodoc.com/presentation_image_h/9fcac2c20e98a062fd11eb0e061bde2e/image-39.jpg)
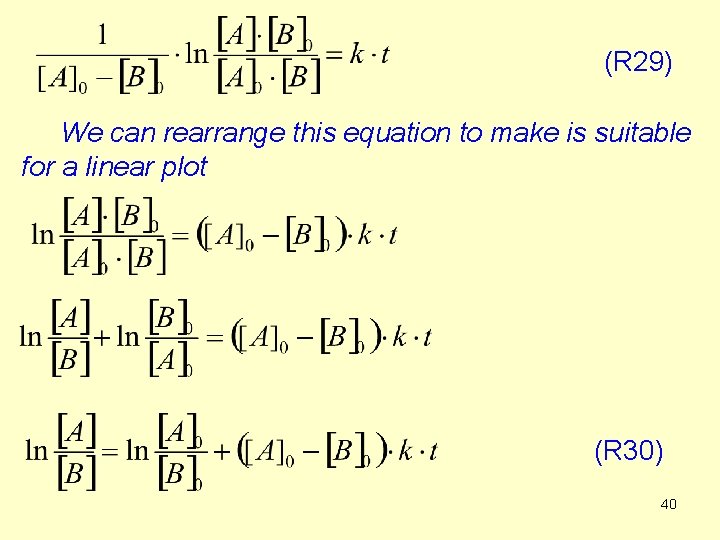
![Plot ln([A]/[B]) as a function of time experimental data a time 41 Plot ln([A]/[B]) as a function of time experimental data a time 41](https://slidetodoc.com/presentation_image_h/9fcac2c20e98a062fd11eb0e061bde2e/image-41.jpg)
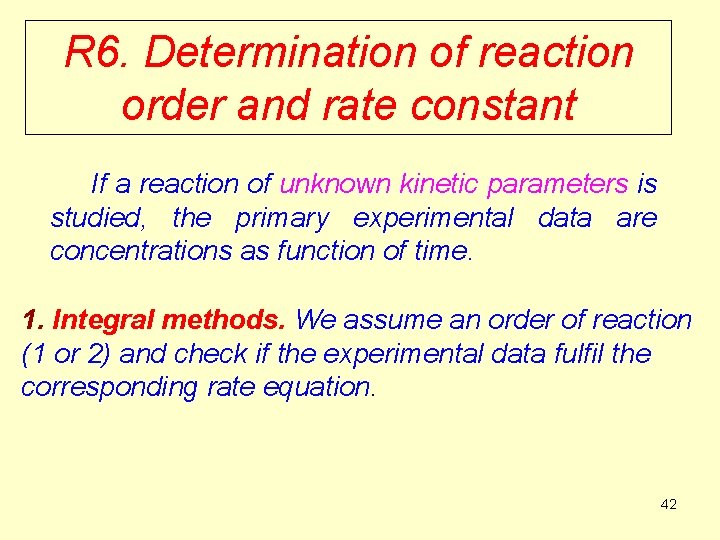
![First order (R 21 b) ln[A] If the experimental data sit on this like First order (R 21 b) ln[A] If the experimental data sit on this like](https://slidetodoc.com/presentation_image_h/9fcac2c20e98a062fd11eb0e061bde2e/image-43.jpg)
![First order (R 21 b) ln[A] If the experimental data do not sit on First order (R 21 b) ln[A] If the experimental data do not sit on](https://slidetodoc.com/presentation_image_h/9fcac2c20e98a062fd11eb0e061bde2e/image-44.jpg)
![Second order (R 26) 1/[A] If the experimental data sit on a this kind Second order (R 26) 1/[A] If the experimental data sit on a this kind](https://slidetodoc.com/presentation_image_h/9fcac2c20e98a062fd11eb0e061bde2e/image-45.jpg)
![Second order (R 26) 1/[A] In this case the experimental data do not sit Second order (R 26) 1/[A] In this case the experimental data do not sit](https://slidetodoc.com/presentation_image_h/9fcac2c20e98a062fd11eb0e061bde2e/image-46.jpg)
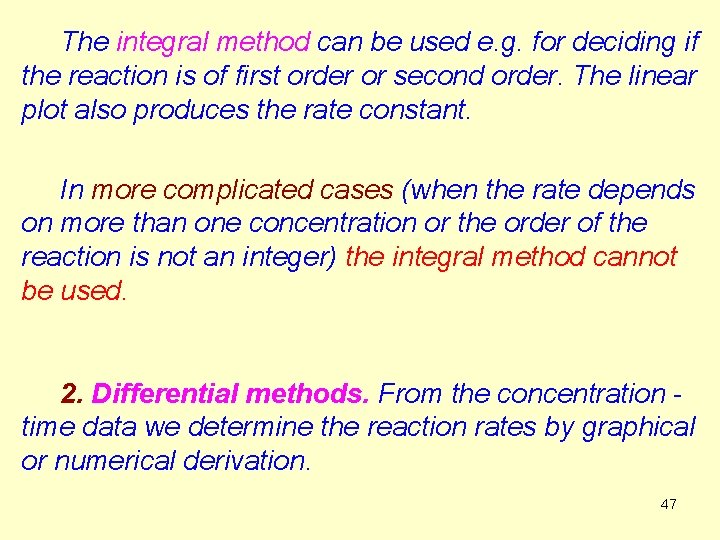
![The concentration of a reactant as a function of time. concentration [A]0 (R 31) The concentration of a reactant as a function of time. concentration [A]0 (R 31)](https://slidetodoc.com/presentation_image_h/9fcac2c20e98a062fd11eb0e061bde2e/image-48.jpg)
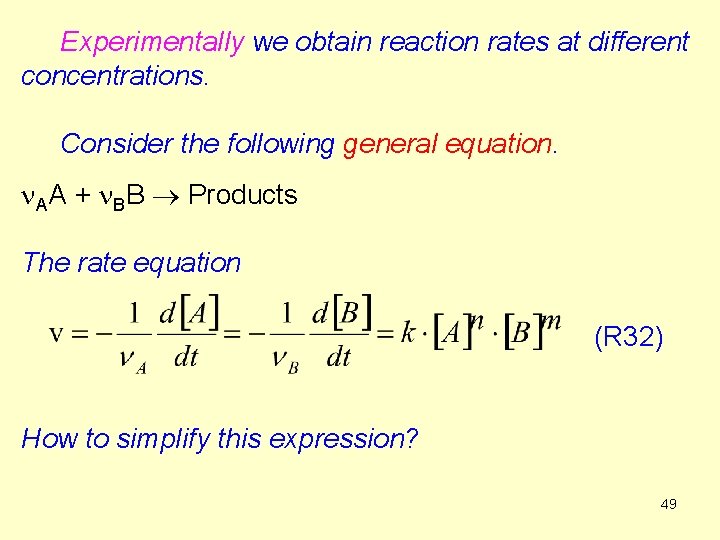
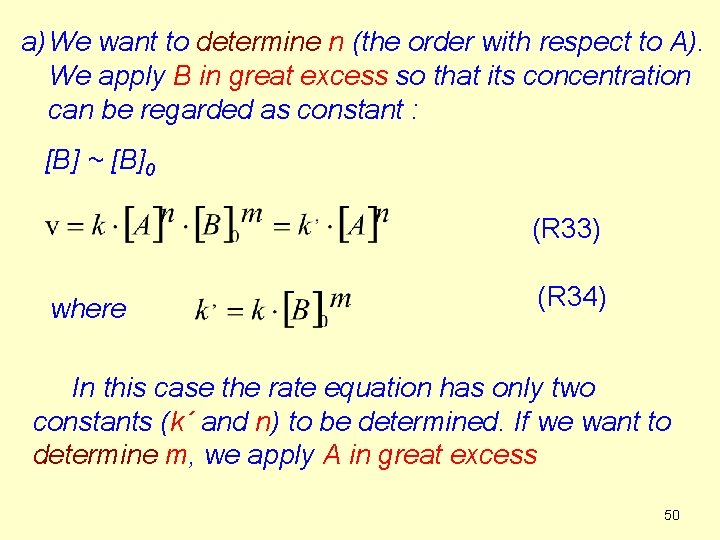
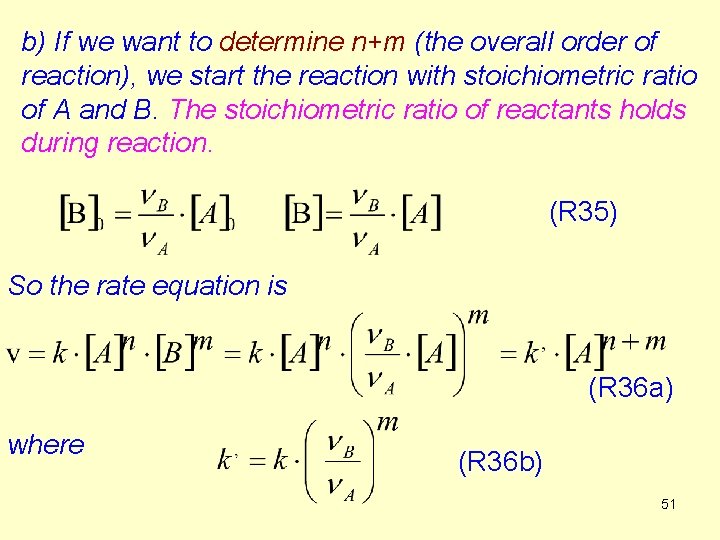
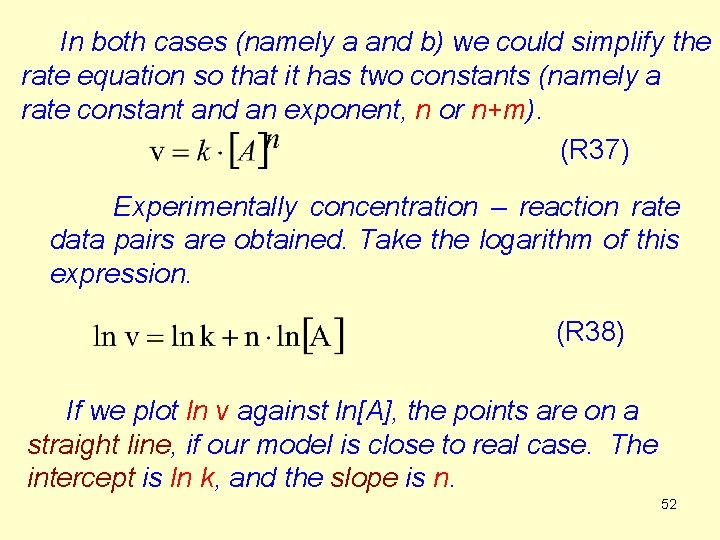
![lnv as a function of ln[A] ln v experimental data a ln k ln[A] lnv as a function of ln[A] ln v experimental data a ln k ln[A]](https://slidetodoc.com/presentation_image_h/9fcac2c20e98a062fd11eb0e061bde2e/image-53.jpg)
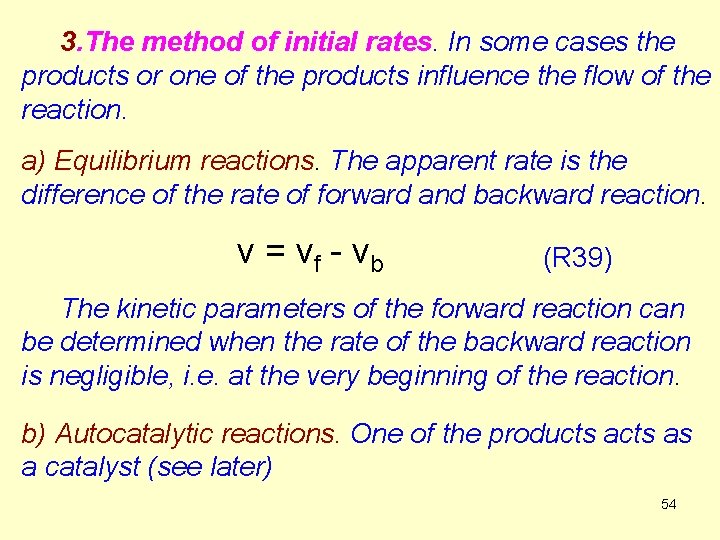
![The concentration of a reactant as a function of time. [A]0 a time 55 The concentration of a reactant as a function of time. [A]0 a time 55](https://slidetodoc.com/presentation_image_h/9fcac2c20e98a062fd11eb0e061bde2e/image-55.jpg)
![The initial rates are determined at various [A]0 concentrations. The kinetic parameters are calculated The initial rates are determined at various [A]0 concentrations. The kinetic parameters are calculated](https://slidetodoc.com/presentation_image_h/9fcac2c20e98a062fd11eb0e061bde2e/image-56.jpg)
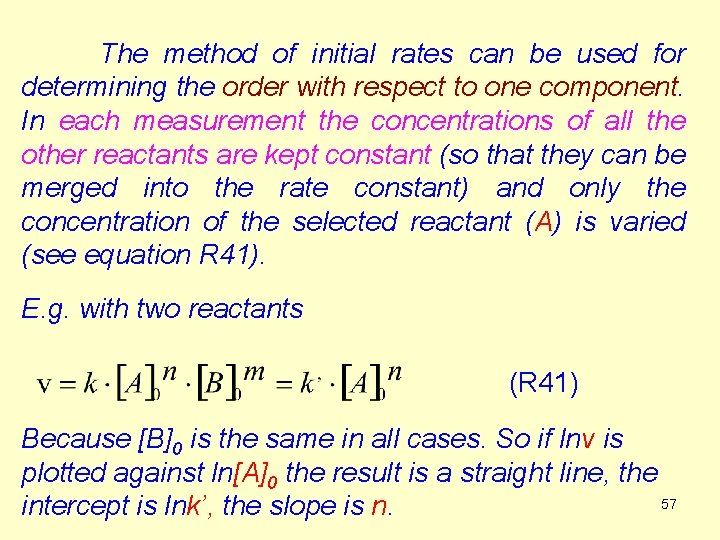
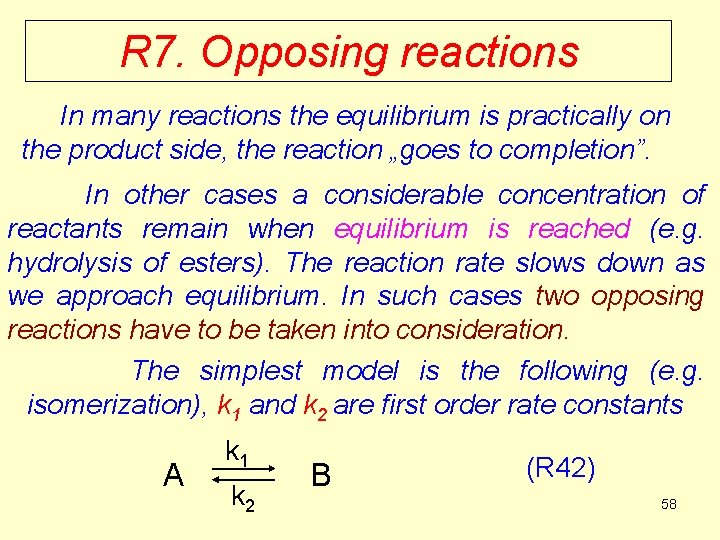
![The rate equation (R 43) At equilibrium v = 0, k 1·[A]e = k The rate equation (R 43) At equilibrium v = 0, k 1·[A]e = k](https://slidetodoc.com/presentation_image_h/9fcac2c20e98a062fd11eb0e061bde2e/image-59.jpg)
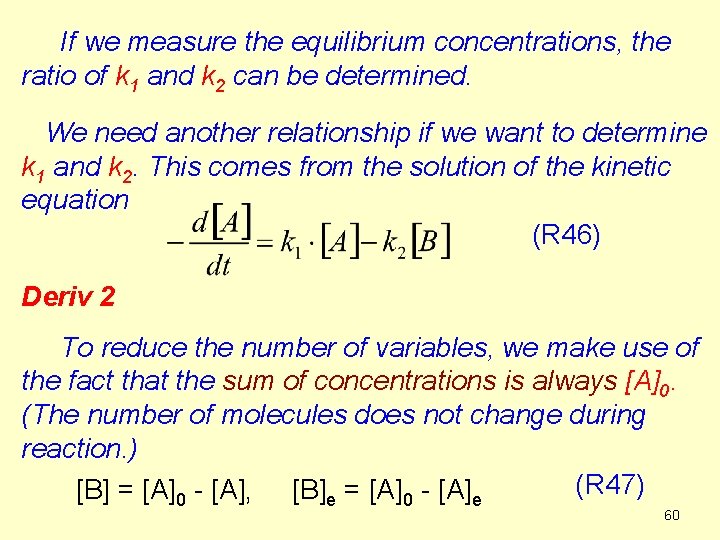
![Before integration we change the last term (k 2·[A]0). 61 Before integration we change the last term (k 2·[A]0). 61](https://slidetodoc.com/presentation_image_h/9fcac2c20e98a062fd11eb0e061bde2e/image-61.jpg)
![We integrate this differential equation from [A]0 to [A] and from 0 to t We integrate this differential equation from [A]0 to [A] and from 0 to t](https://slidetodoc.com/presentation_image_h/9fcac2c20e98a062fd11eb0e061bde2e/image-62.jpg)
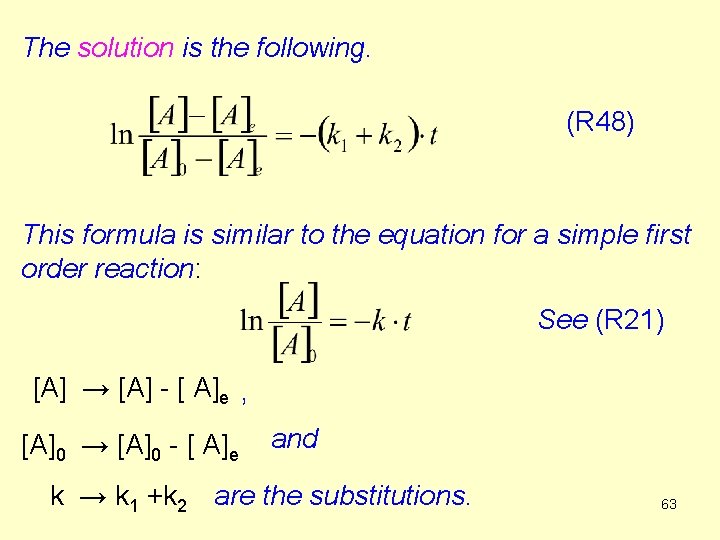
![We can determine k 1+k 2 if we plot ln([A]-[A]0) against time. ln([A]-[A]e) experimental We can determine k 1+k 2 if we plot ln([A]-[A]0) against time. ln([A]-[A]e) experimental](https://slidetodoc.com/presentation_image_h/9fcac2c20e98a062fd11eb0e061bde2e/image-64.jpg)
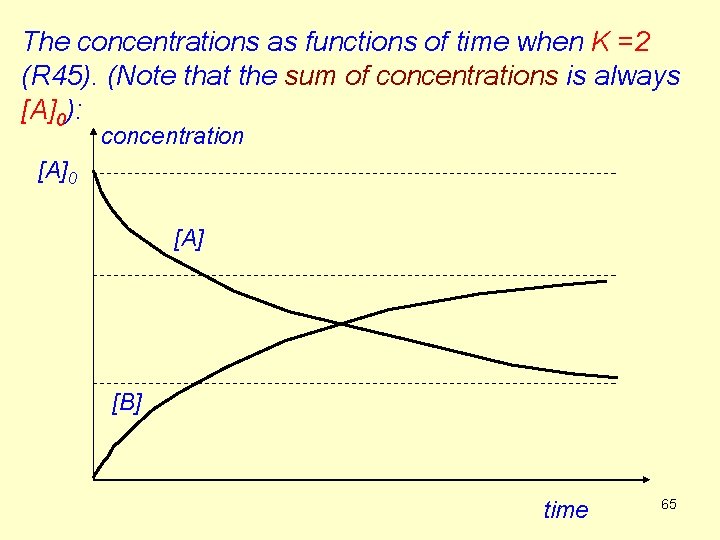
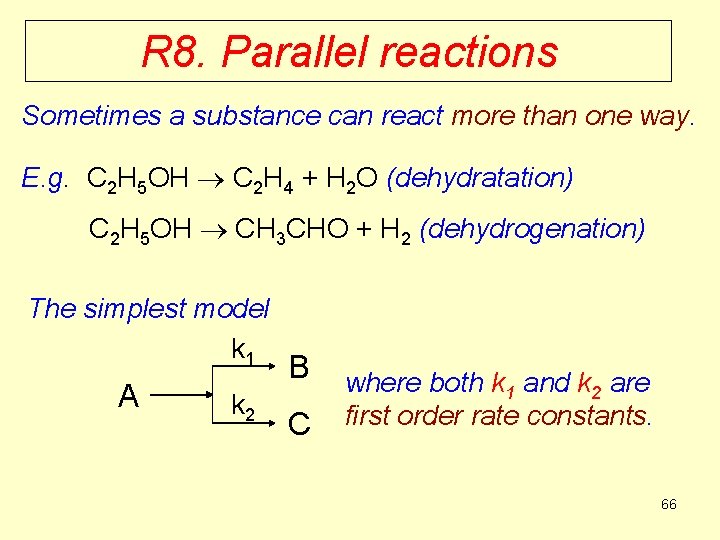
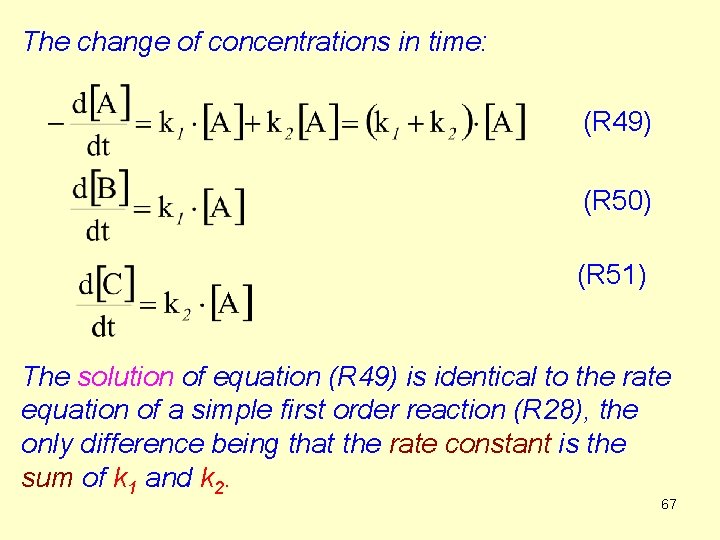
![Time dependence of the concentration of A (R 52) ln[A] is a linear Time dependence of the concentration of A (R 52) ln[A] is a linear](https://slidetodoc.com/presentation_image_h/9fcac2c20e98a062fd11eb0e061bde2e/image-68.jpg)
![Linear plot ln[A] experimental data ln[A]0 tga = -(k 1+k 2) a time 69 Linear plot ln[A] experimental data ln[A]0 tga = -(k 1+k 2) a time 69](https://slidetodoc.com/presentation_image_h/9fcac2c20e98a062fd11eb0e061bde2e/image-69.jpg)
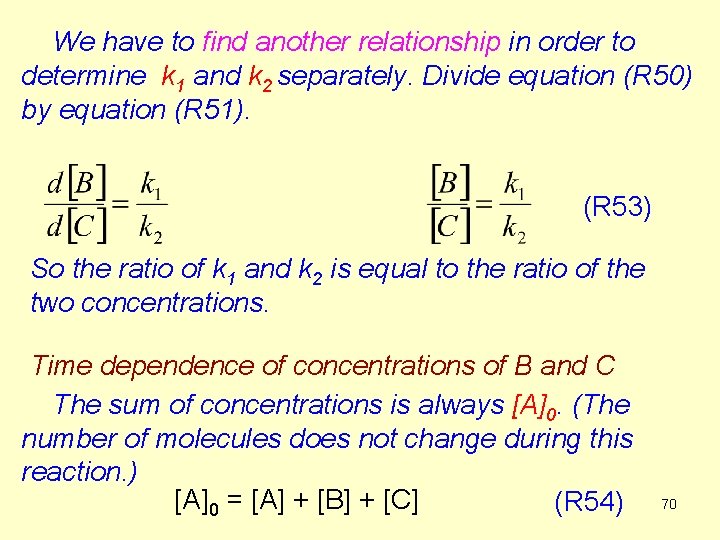
![Using equation (R 52) If we want the time dependence of [B], eliminate [C] Using equation (R 52) If we want the time dependence of [B], eliminate [C]](https://slidetodoc.com/presentation_image_h/9fcac2c20e98a062fd11eb0e061bde2e/image-71.jpg)
![Results: (R 55) By similar consideration the time dependence of [C] (R 56) The Results: (R 55) By similar consideration the time dependence of [C] (R 56) The](https://slidetodoc.com/presentation_image_h/9fcac2c20e98a062fd11eb0e061bde2e/image-72.jpg)
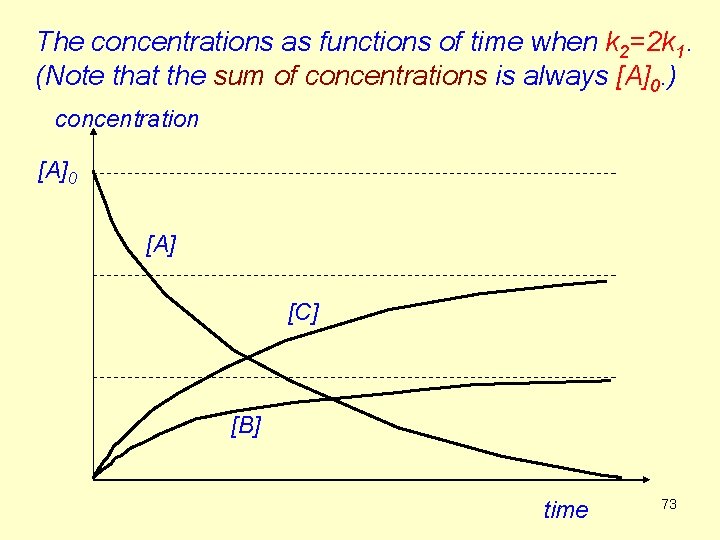
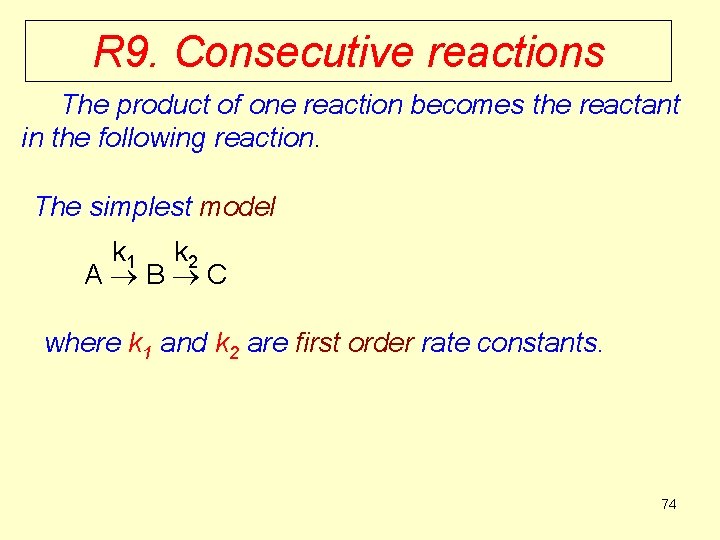
![Substituting the right hand side of this expression for [A] into equation R 57 Substituting the right hand side of this expression for [A] into equation R 57](https://slidetodoc.com/presentation_image_h/9fcac2c20e98a062fd11eb0e061bde2e/image-75.jpg)
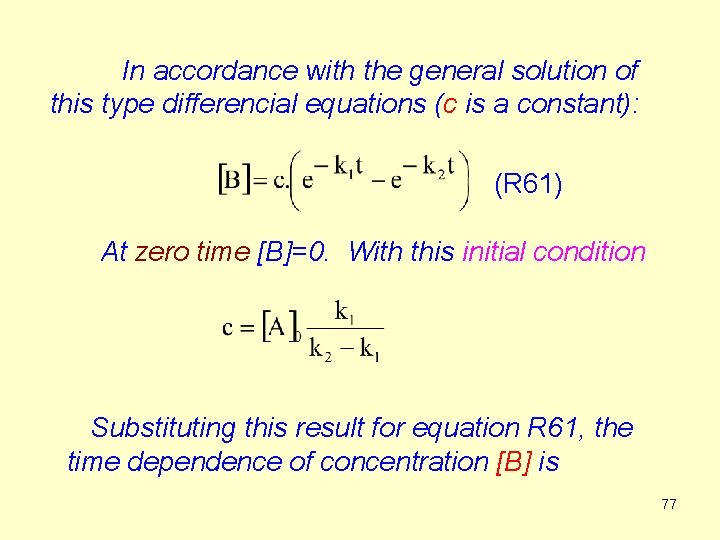
![(R 62) The concentration of A decreases exponentionally. [B] reaches a maximum, then decreases (R 62) The concentration of A decreases exponentionally. [B] reaches a maximum, then decreases](https://slidetodoc.com/presentation_image_h/9fcac2c20e98a062fd11eb0e061bde2e/image-77.jpg)
![The concentrations as functions of time. concentration [A]0 [A] [C] [B] tm time 79 The concentrations as functions of time. concentration [A]0 [A] [C] [B] tm time 79](https://slidetodoc.com/presentation_image_h/9fcac2c20e98a062fd11eb0e061bde2e/image-78.jpg)
![How to find the maximum of the [B] – t curve (when [B] is How to find the maximum of the [B] – t curve (when [B] is](https://slidetodoc.com/presentation_image_h/9fcac2c20e98a062fd11eb0e061bde2e/image-79.jpg)
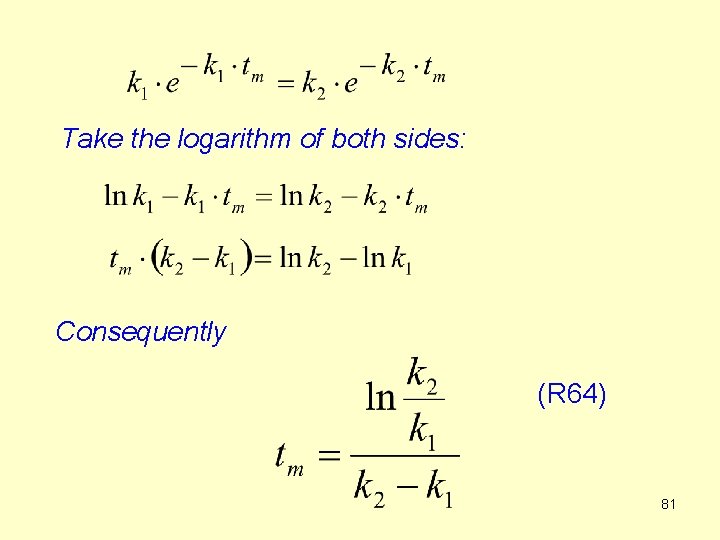
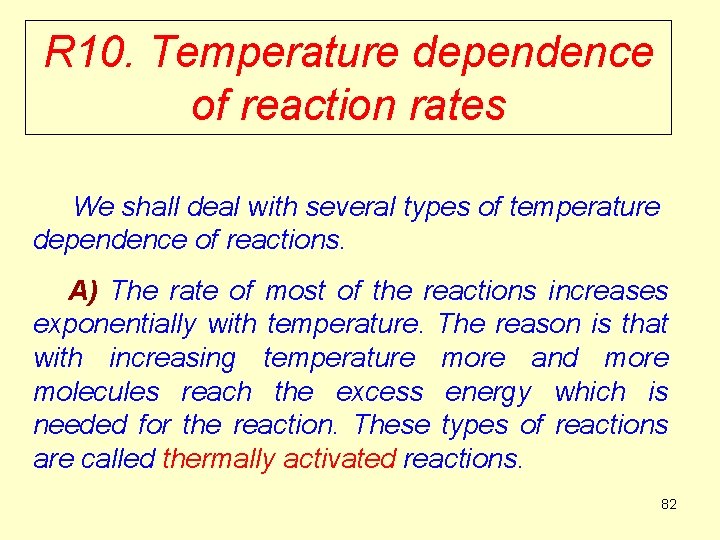
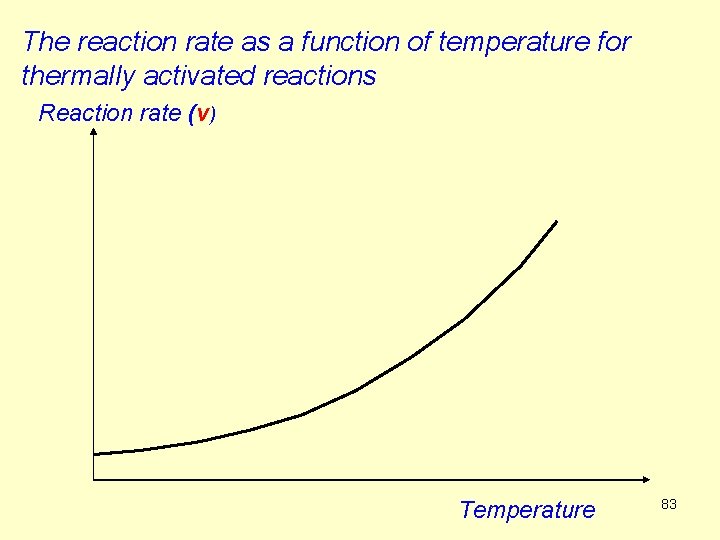
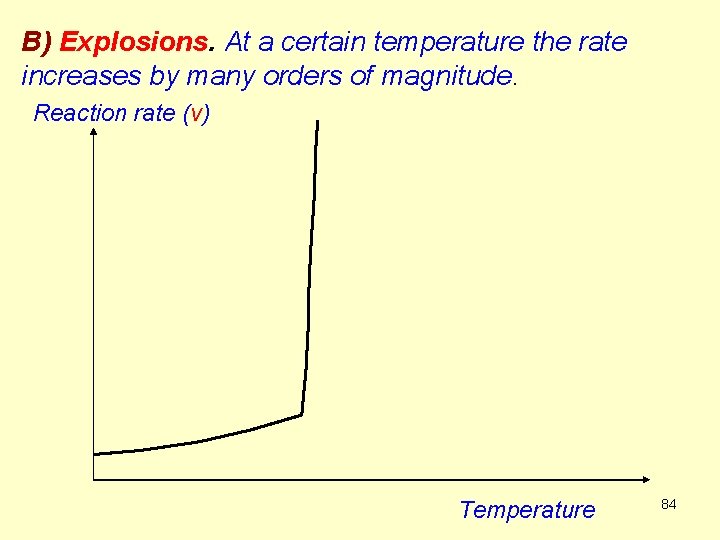
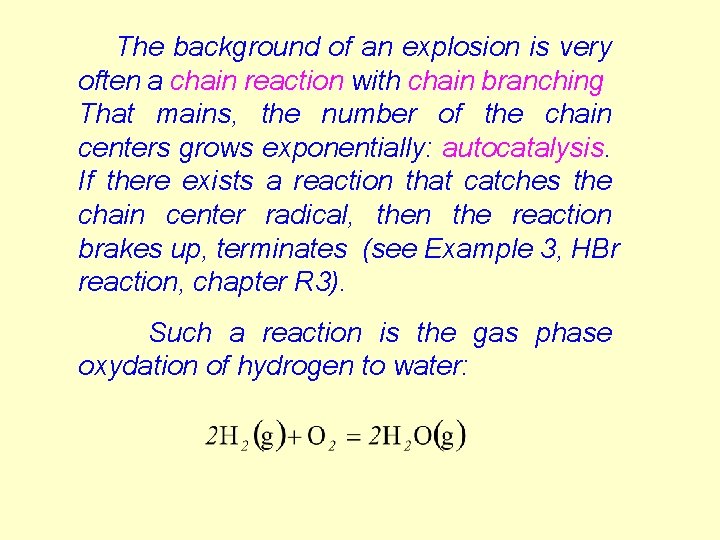
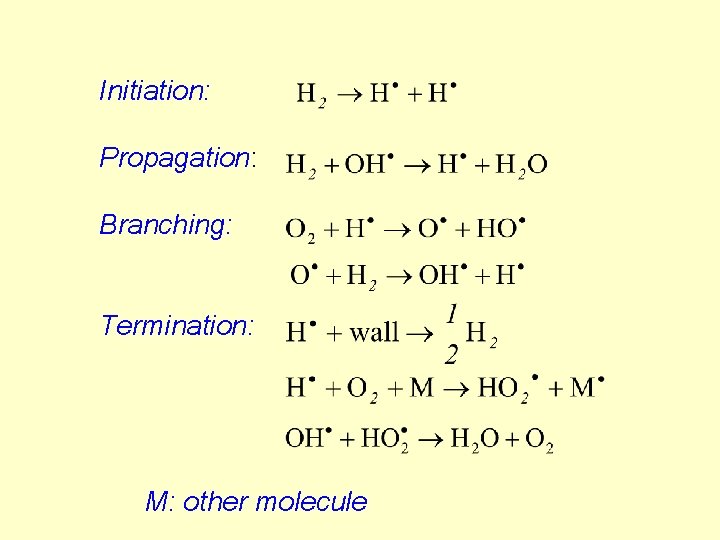
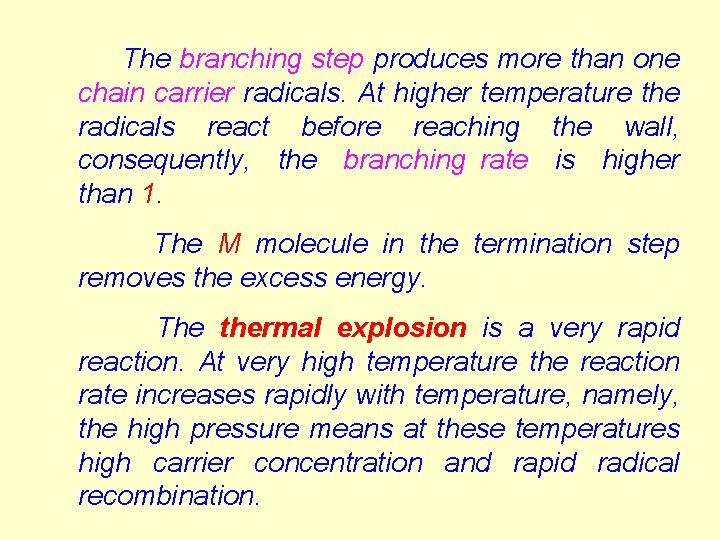
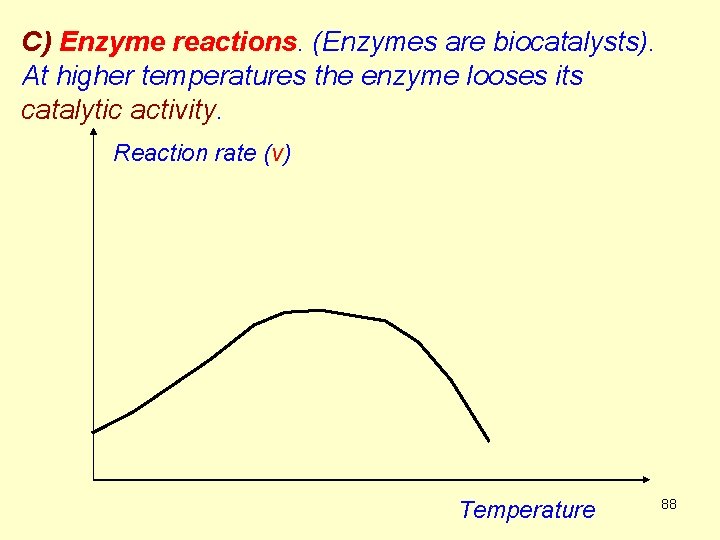
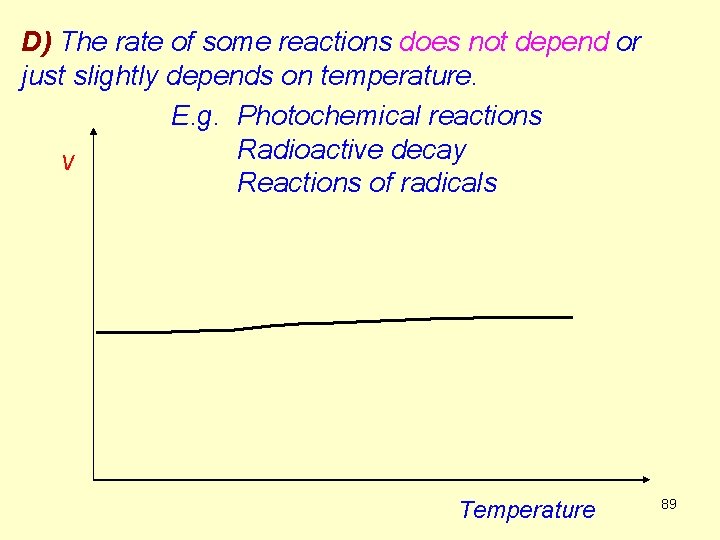
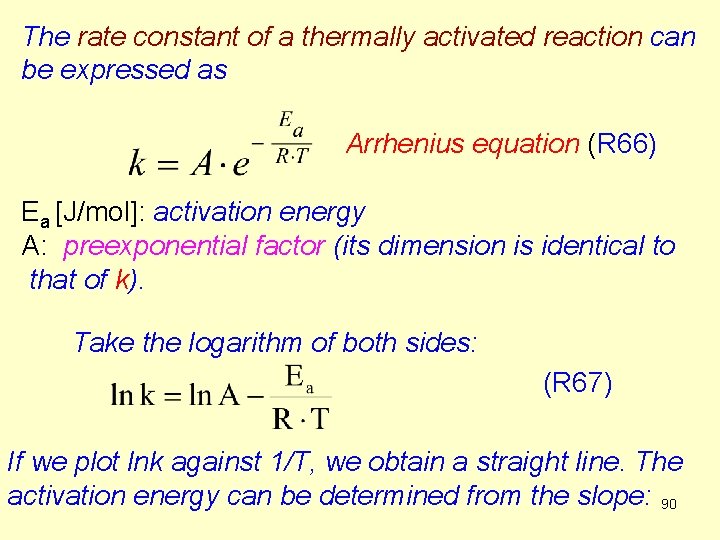
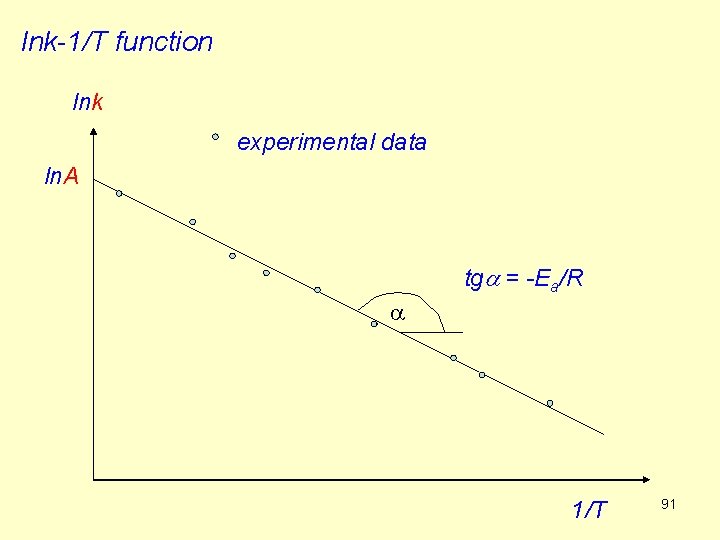
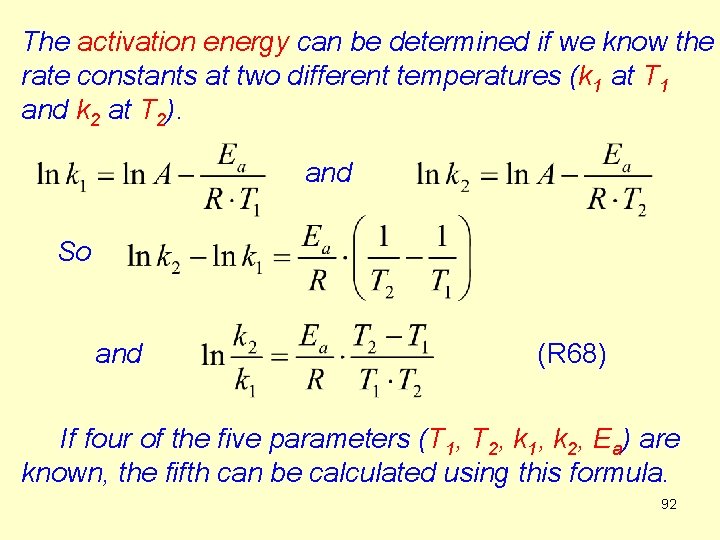
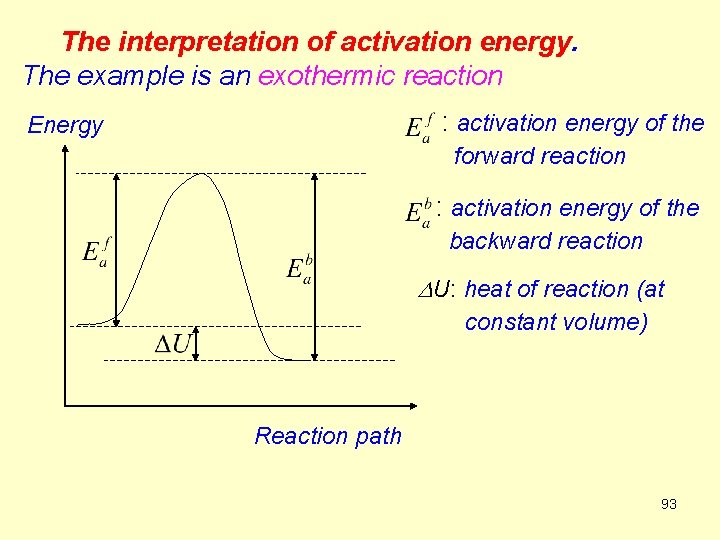
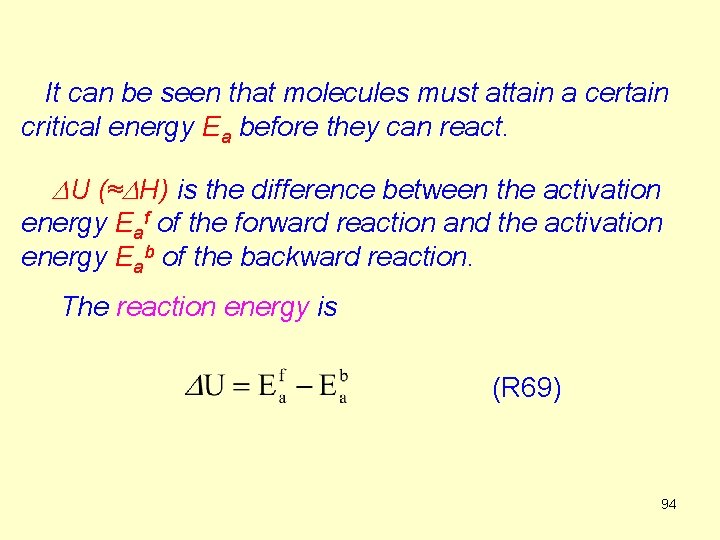
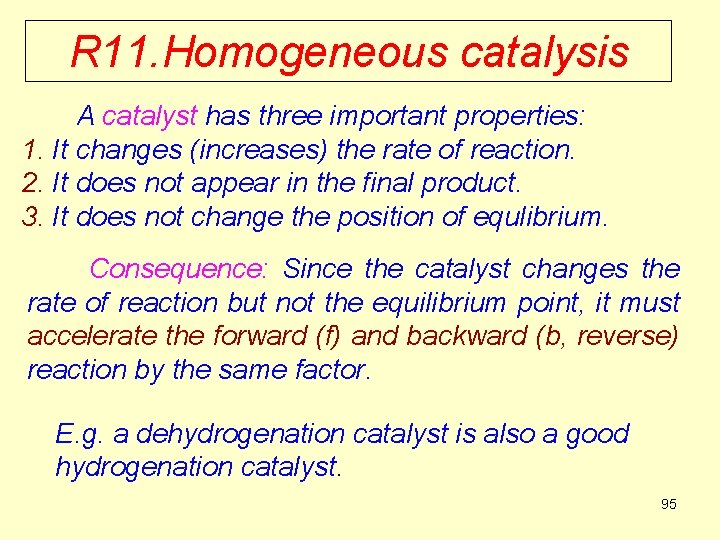
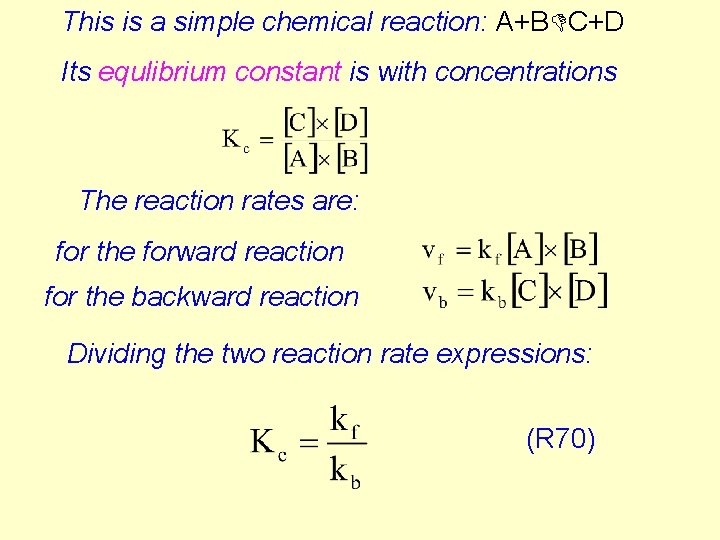
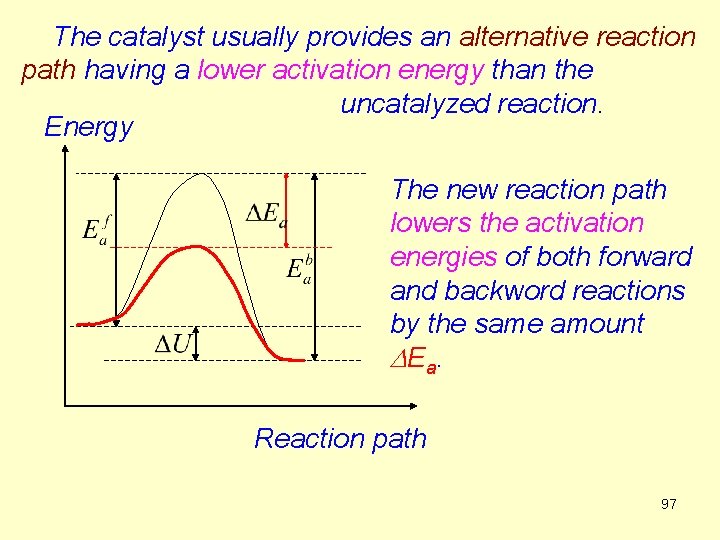
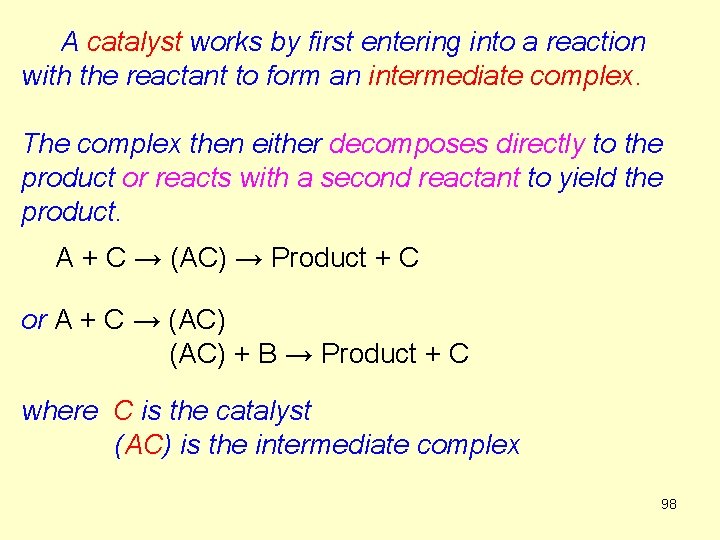
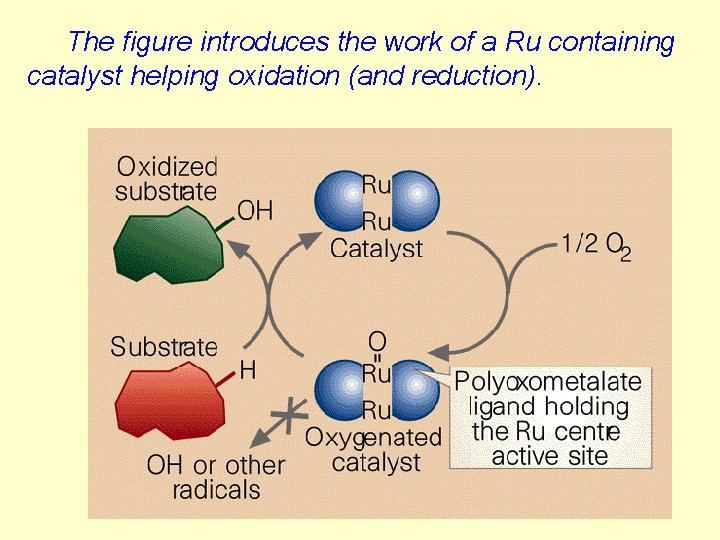
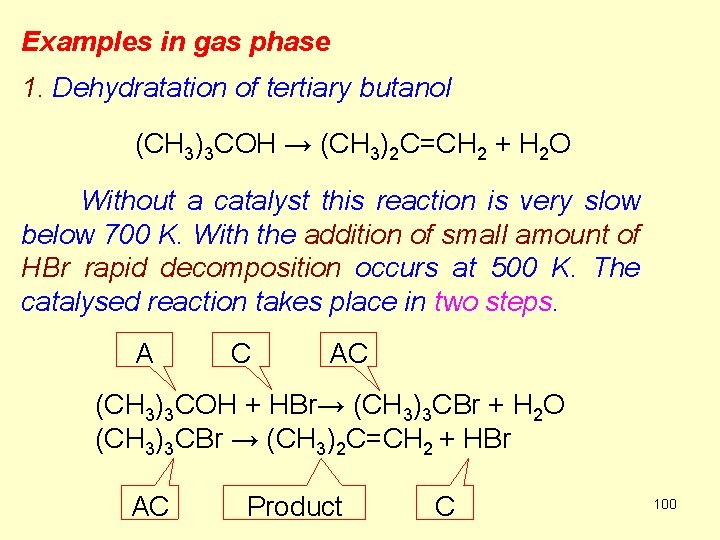
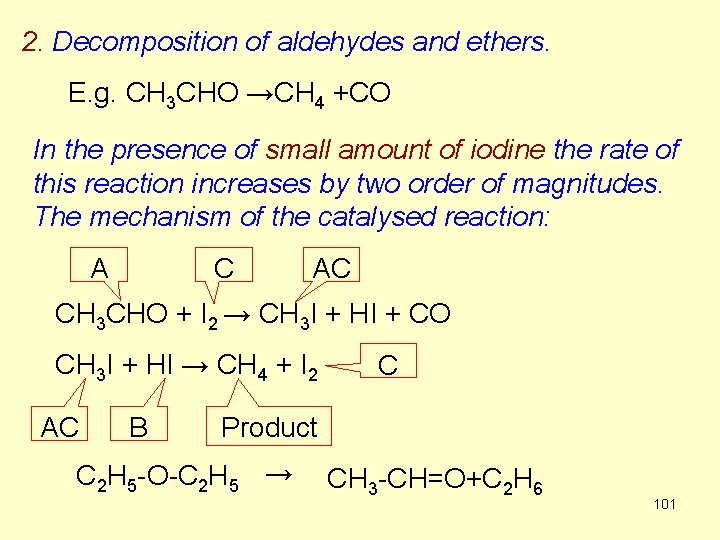
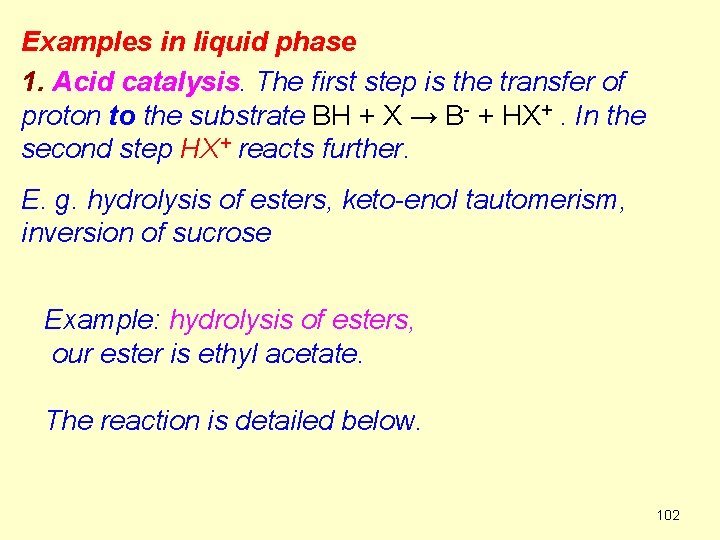
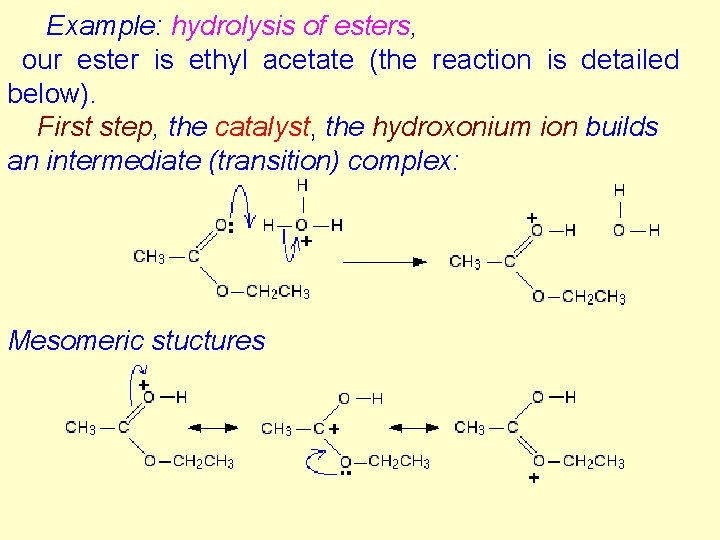
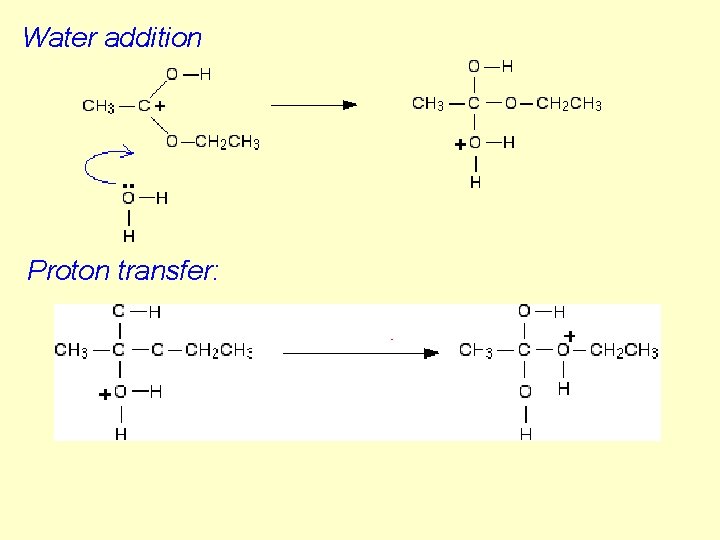
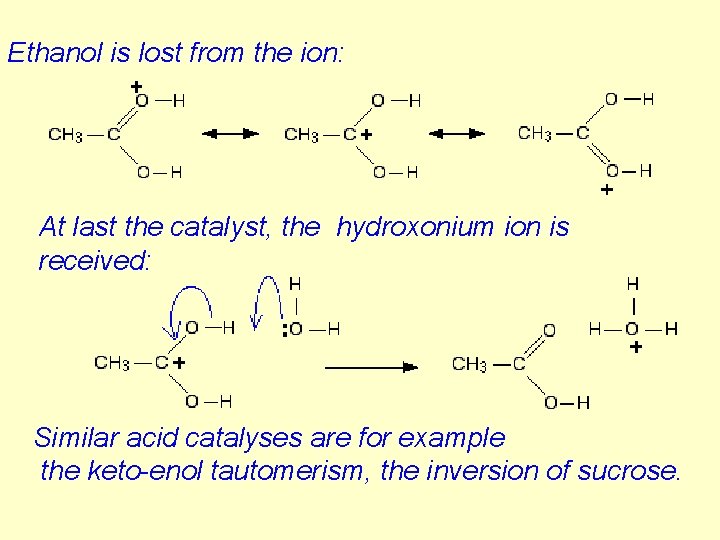
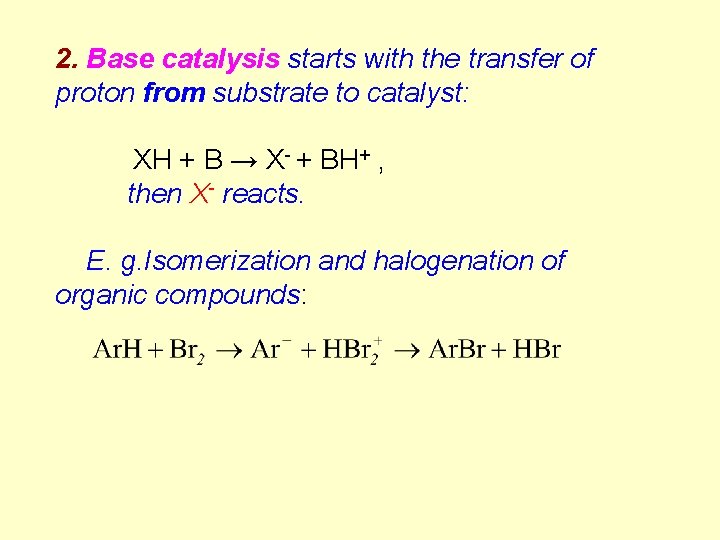
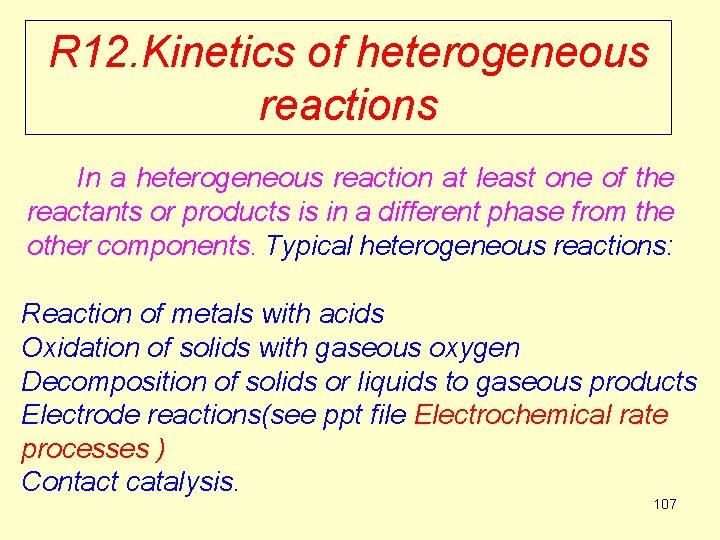
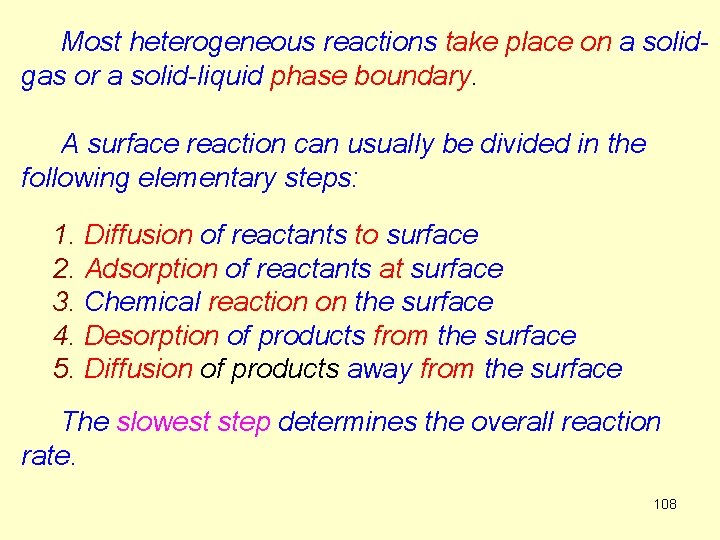
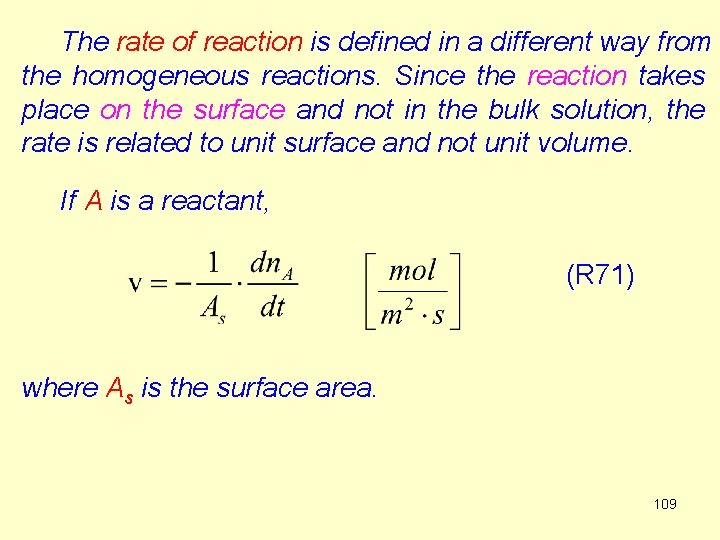
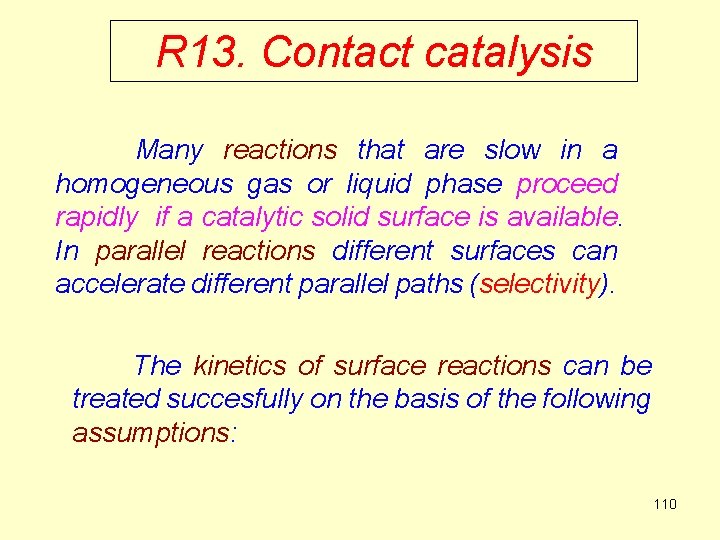
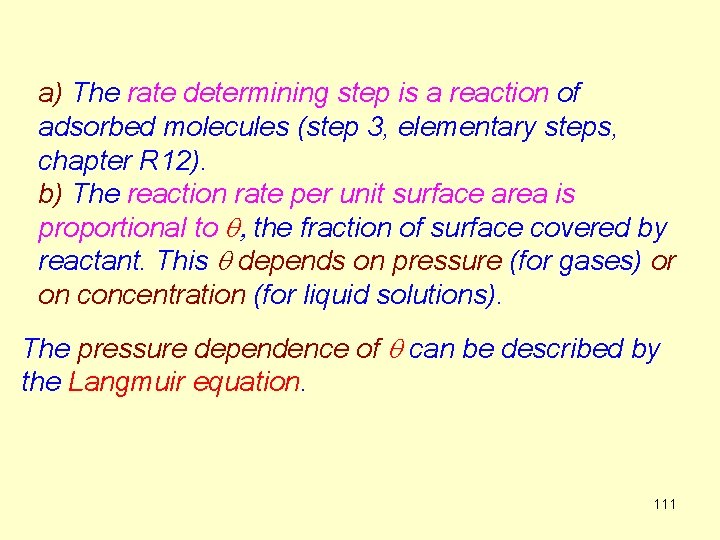
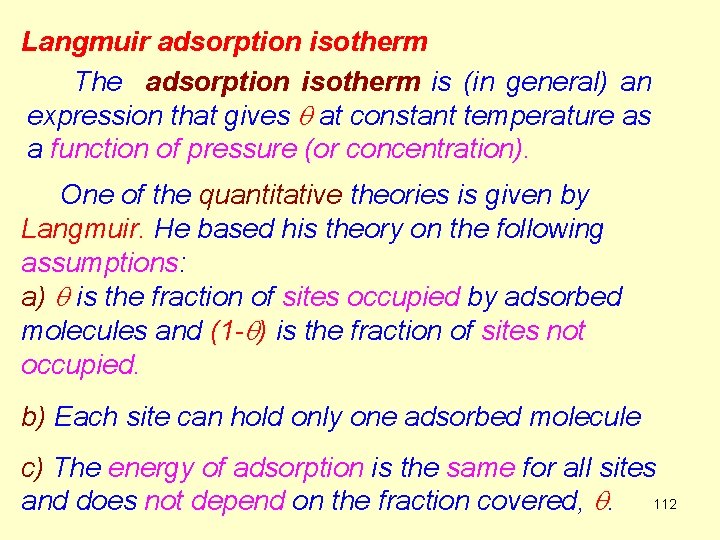
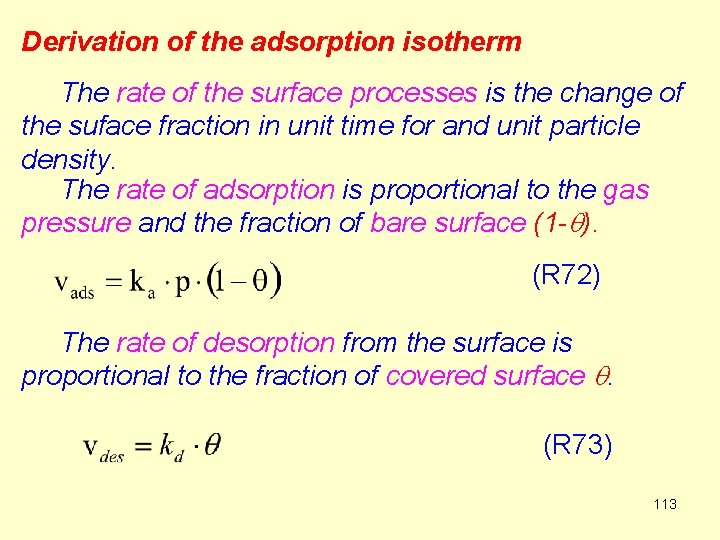
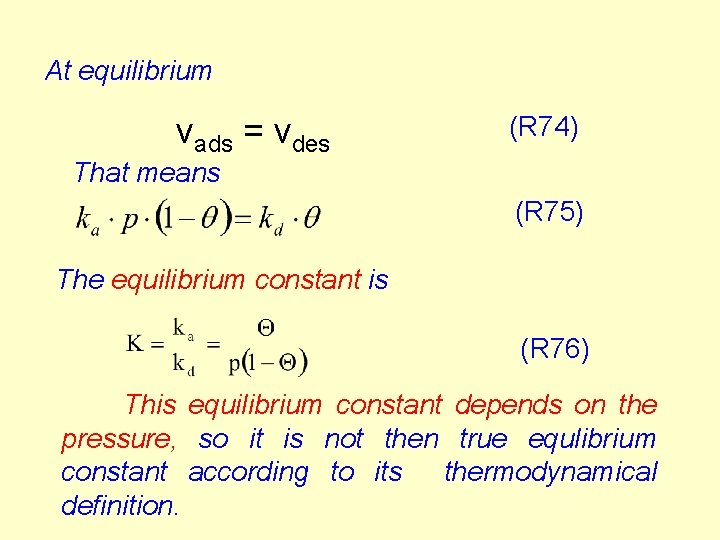
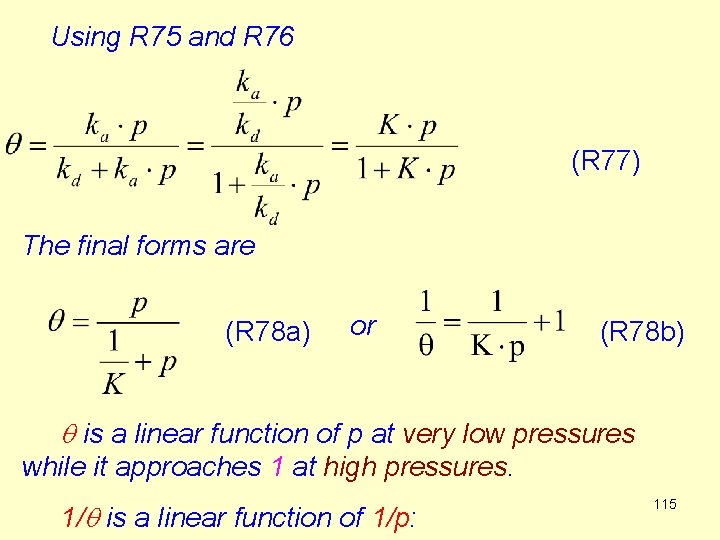
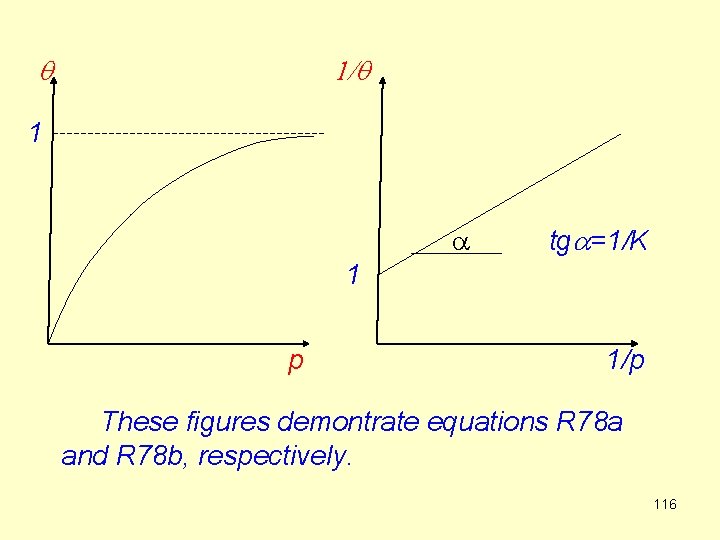
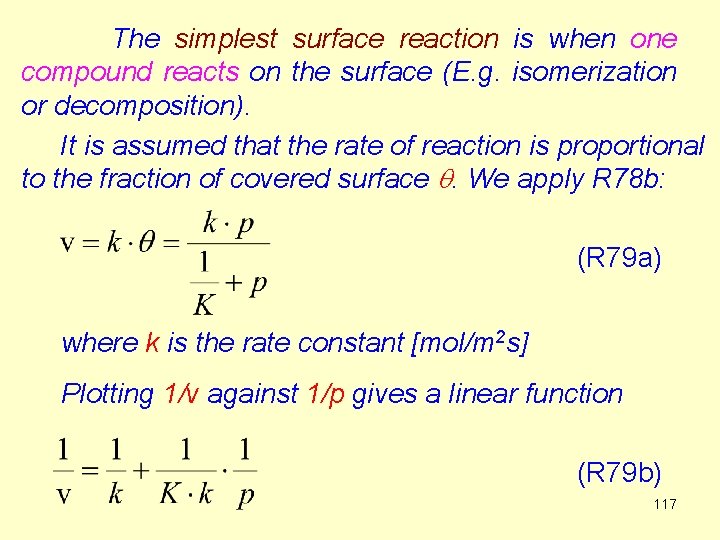
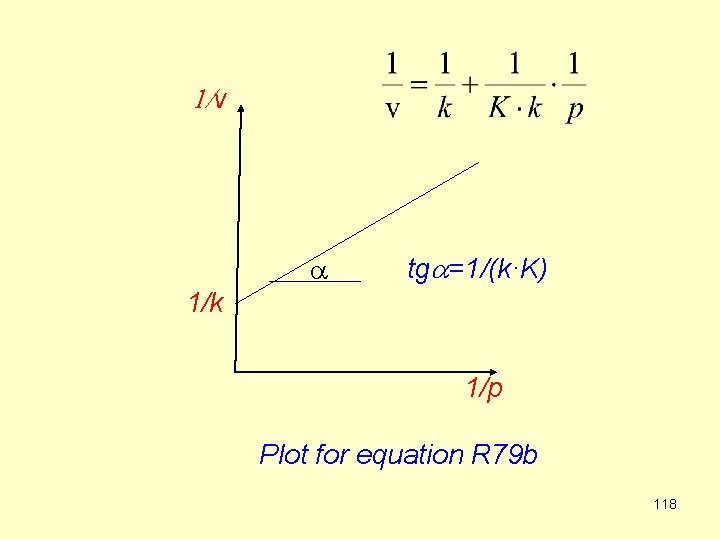
- Slides: 117
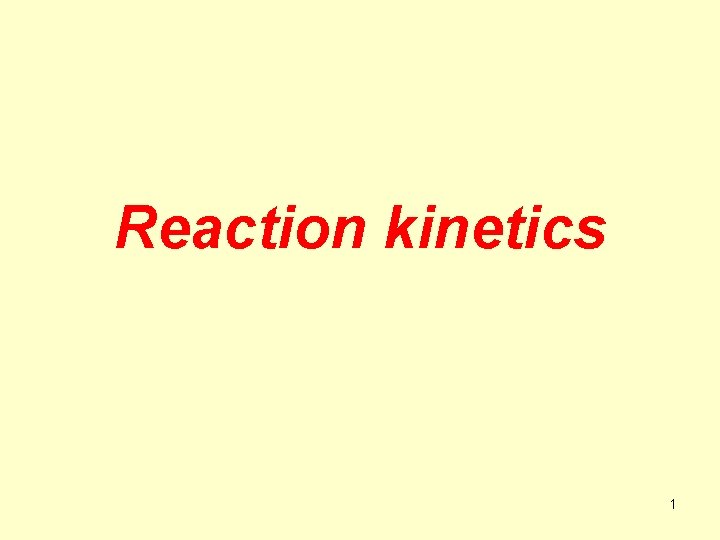
Reaction kinetics 1
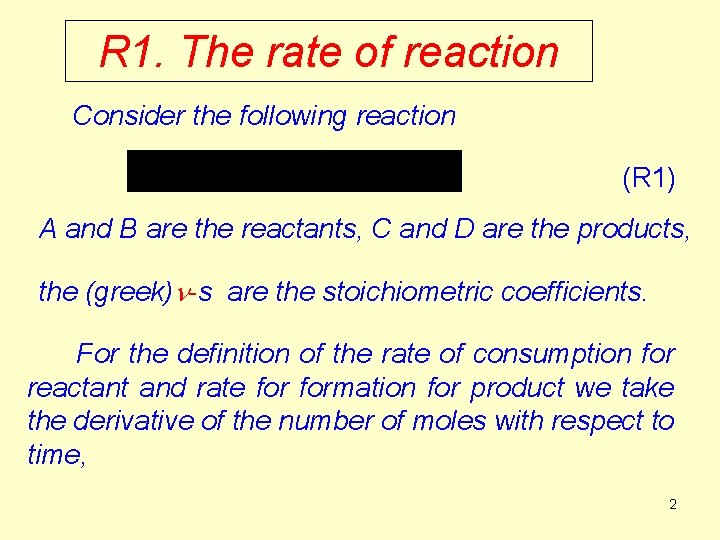
R 1. The rate of reaction Consider the following reaction (R 1) A and B are the reactants, C and D are the products, the (greek)n-s are the stoichiometric coefficients. For the definition of the rate of consumption for reactant and rate formation for product we take the derivative of the number of moles with respect to time, 2
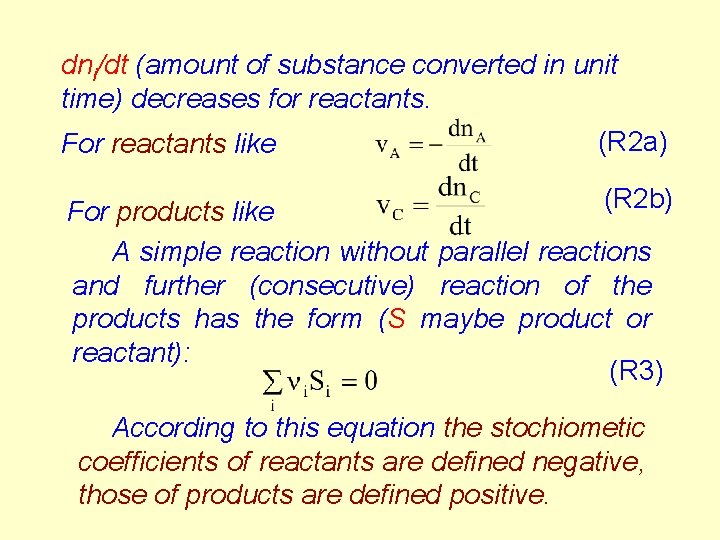
dni/dt (amount of substance converted in unit time) decreases for reactants. For reactants like (R 2 a) (R 2 b) For products like A simple reaction without parallel reactions and further (consecutive) reaction of the products has the form (S maybe product or reactant): (R 3) According to this equation the stochiometic coefficients of reactants are defined negative, those of products are defined positive.
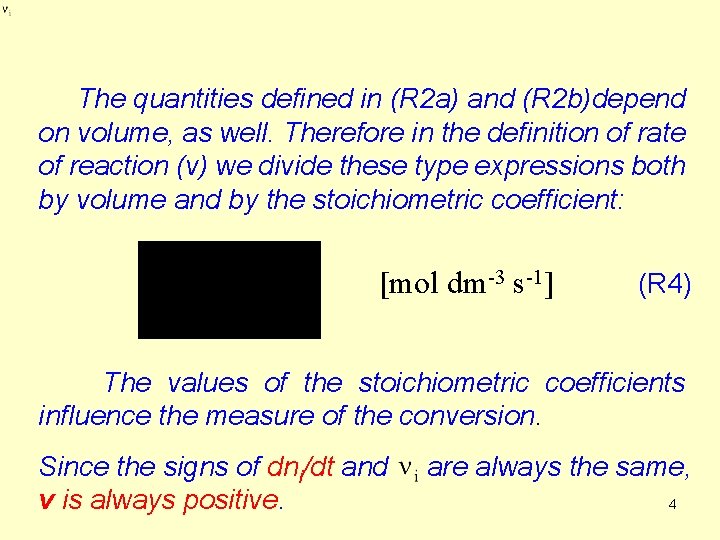
The quantities defined in (R 2 a) and (R 2 b)depend on volume, as well. Therefore in the definition of rate of reaction (v) we divide these type expressions both by volume and by the stoichiometric coefficient: [mol dm-3 s-1] (R 4) The values of the stoichiometric coefficients influence the measure of the conversion. Since the signs of dni/dt and are always the same, 4 v is always positive.
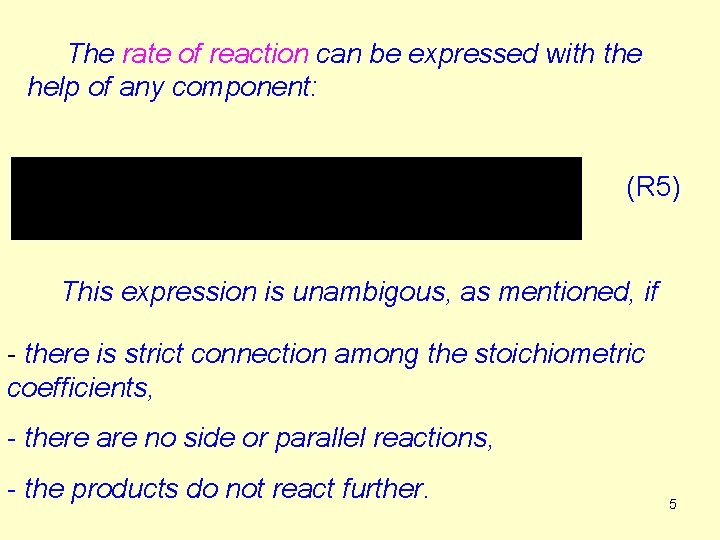
The rate of reaction can be expressed with the help of any component: (R 5) This expression is unambigous, as mentioned, if - there is strict connection among the stoichiometric coefficients, - there are no side or parallel reactions, - the products do not react further. 5
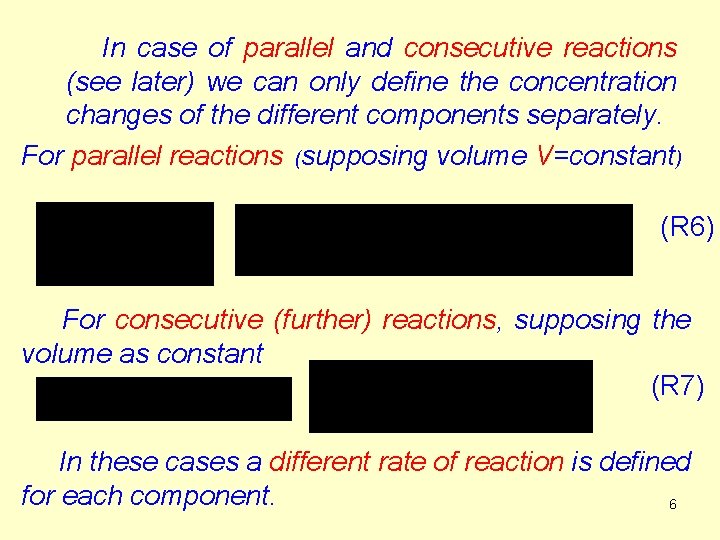
In case of parallel and consecutive reactions (see later) we can only define the concentration changes of the different components separately. For parallel reactions (supposing volume V=constant) (R 6) For consecutive (further) reactions, supposing the volume as constant (R 7) In these cases a different rate of reaction is defined for each component. 6
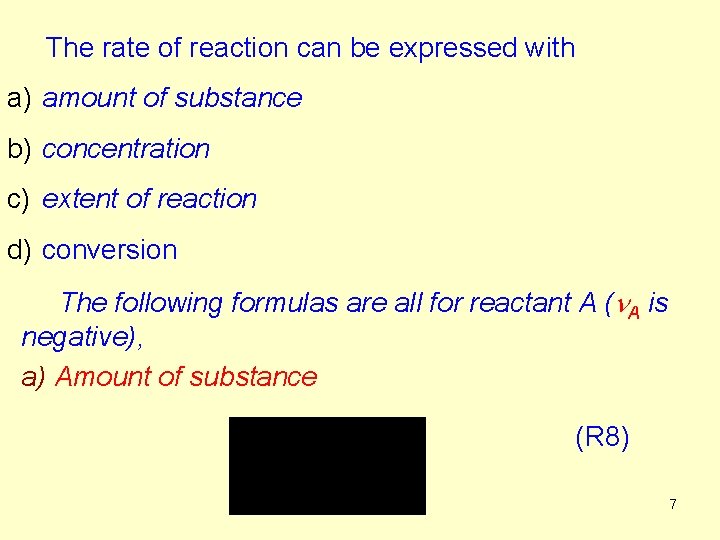
The rate of reaction can be expressed with a) amount of substance b) concentration c) extent of reaction d) conversion The following formulas are all for reactant A (n. A is negative), a) Amount of substance (R 8) 7
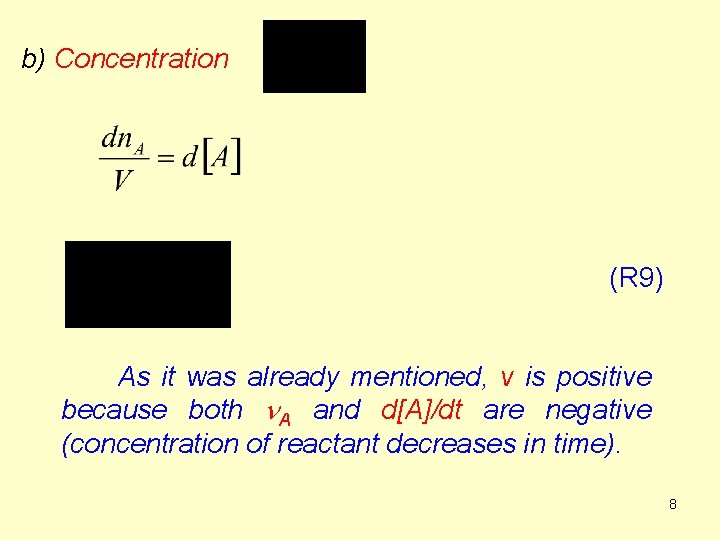
b) Concentration (R 9) As it was already mentioned, v is positive because both n. A and d[A]/dt are negative (concentration of reactant decreases in time). 8
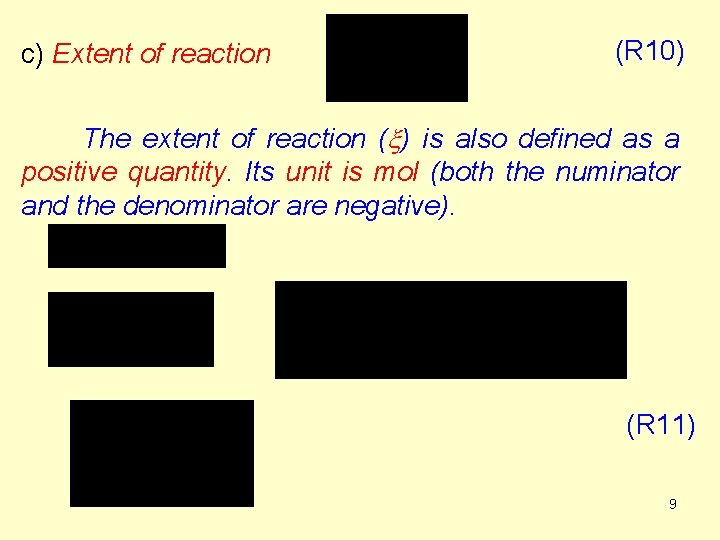
c) Extent of reaction (R 10) The extent of reaction (x) is also defined as a positive quantity. Its unit is mol (both the numinator and the denominator are negative). (R 11) 9
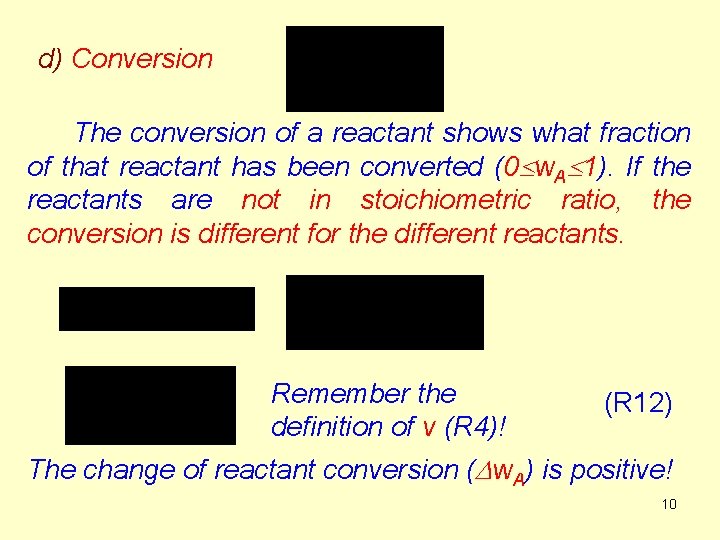
d) Conversion The conversion of a reactant shows what fraction of that reactant has been converted (0 w. A 1). If the reactants are not in stoichiometric ratio, the conversion is different for the different reactants. Remember the definition of v (R 4)! (R 12) The change of reactant conversion (Dw. A) is positive! 10
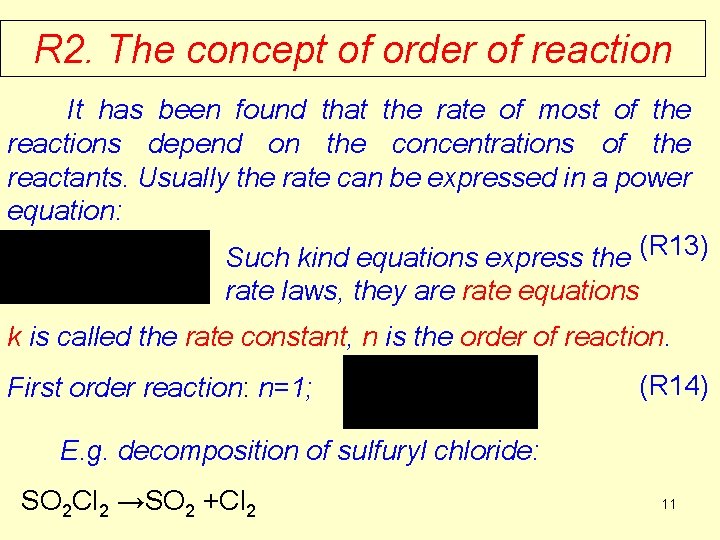
R 2. The concept of order of reaction It has been found that the rate of most of the reactions depend on the concentrations of the reactants. Usually the rate can be expressed in a power equation: Such kind equations express the (R 13) rate laws, they are rate equations k is called the rate constant, n is the order of reaction. First order reaction: n=1; (R 14) E. g. decomposition of sulfuryl chloride: SO 2 Cl 2 →SO 2 +Cl 2 11
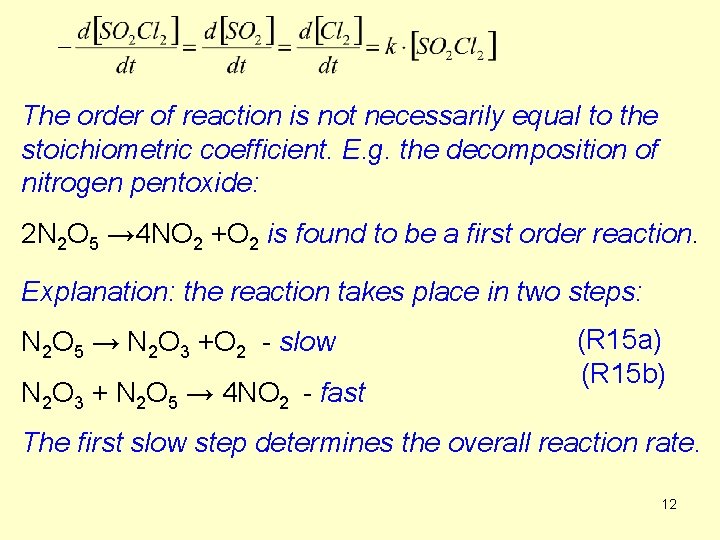
The order of reaction is not necessarily equal to the stoichiometric coefficient. E. g. the decomposition of nitrogen pentoxide: 2 N 2 O 5 → 4 NO 2 +O 2 is found to be a first order reaction. Explanation: the reaction takes place in two steps: N 2 O 5 → N 2 O 3 +O 2 - slow N 2 O 3 + N 2 O 5 → 4 NO 2 - fast (R 15 a) (R 15 b) The first slow step determines the overall reaction rate. 12
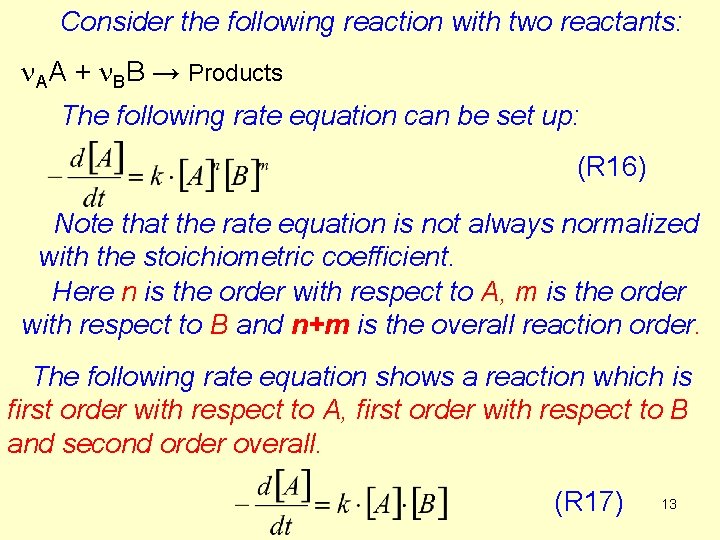
Consider the following reaction with two reactants: n. AA + n. BB → Products The following rate equation can be set up: (R 16) Note that the rate equation is not always normalized with the stoichiometric coefficient. Here n is the order with respect to A, m is the order with respect to B and n+m is the overall reaction order. The following rate equation shows a reaction which is first order with respect to A, first order with respect to B and second order overall. (R 17) 13
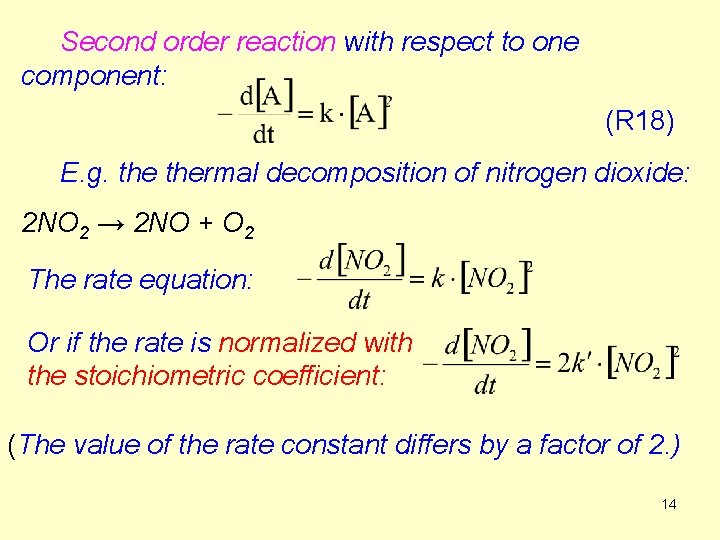
Second order reaction with respect to one component: (R 18) E. g. thermal decomposition of nitrogen dioxide: 2 NO 2 → 2 NO + O 2 The rate equation: Or if the rate is normalized with the stoichiometric coefficient: (The value of the rate constant differs by a factor of 2. ) 14
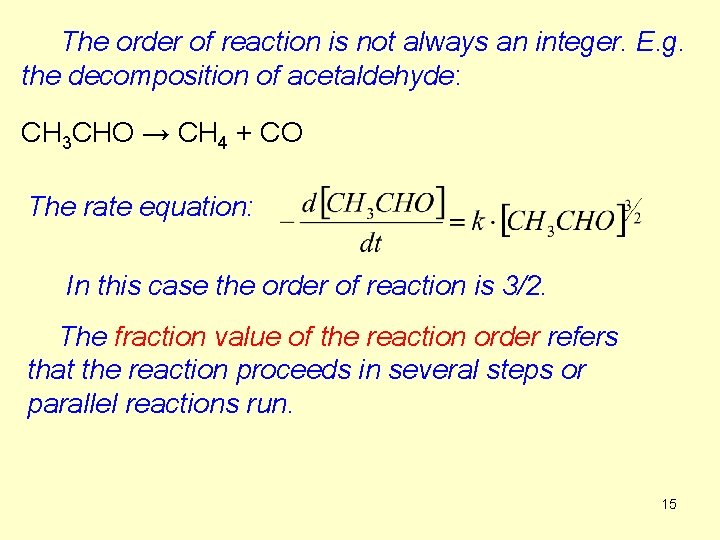
The order of reaction is not always an integer. E. g. the decomposition of acetaldehyde: CH 3 CHO → CH 4 + CO The rate equation: In this case the order of reaction is 3/2. The fraction value of the reaction order refers that the reaction proceeds in several steps or parallel reactions run. 15
![The unit of rate constant depends on the order of reaction First order k The unit of rate constant depends on the order of reaction. First order [k]](https://slidetodoc.com/presentation_image_h/9fcac2c20e98a062fd11eb0e061bde2e/image-16.jpg)
The unit of rate constant depends on the order of reaction. First order [k] = s-1 Second order [k] = dm 3 mol-1 s-1 n order [k] = (time)-1 (concentration)1 -n 16
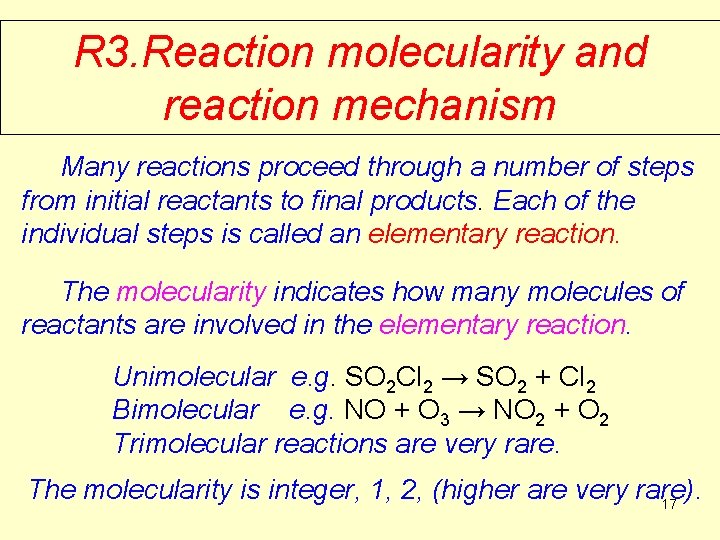
R 3. Reaction molecularity and reaction mechanism Many reactions proceed through a number of steps from initial reactants to final products. Each of the individual steps is called an elementary reaction. The molecularity indicates how many molecules of reactants are involved in the elementary reaction. Unimolecular e. g. SO 2 Cl 2 → SO 2 + Cl 2 Bimolecular e. g. NO + O 3 → NO 2 + O 2 Trimolecular reactions are very rare. The molecularity is integer, 1, 2, (higher are very rare). 17
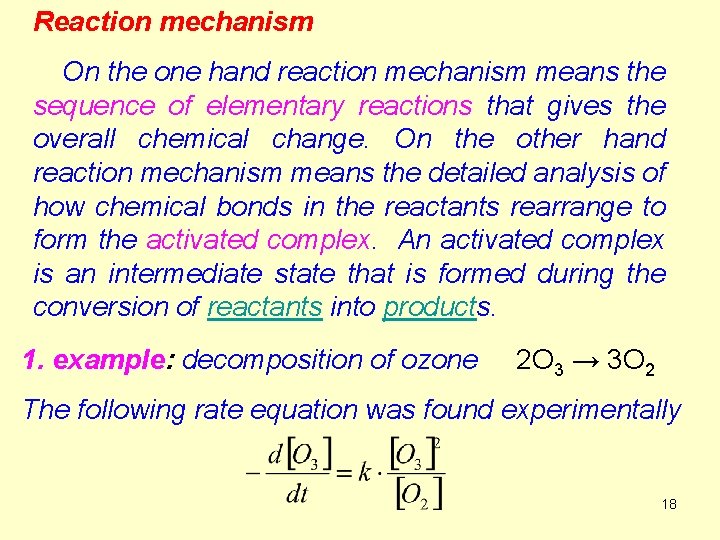
Reaction mechanism On the one hand reaction mechanism means the sequence of elementary reactions that gives the overall chemical change. On the other hand reaction mechanism means the detailed analysis of how chemical bonds in the reactants rearrange to form the activated complex. An activated complex is an intermediate state that is formed during the conversion of reactants into products. 1. example: decomposition of ozone 2 O 3 → 3 O 2 The following rate equation was found experimentally 18
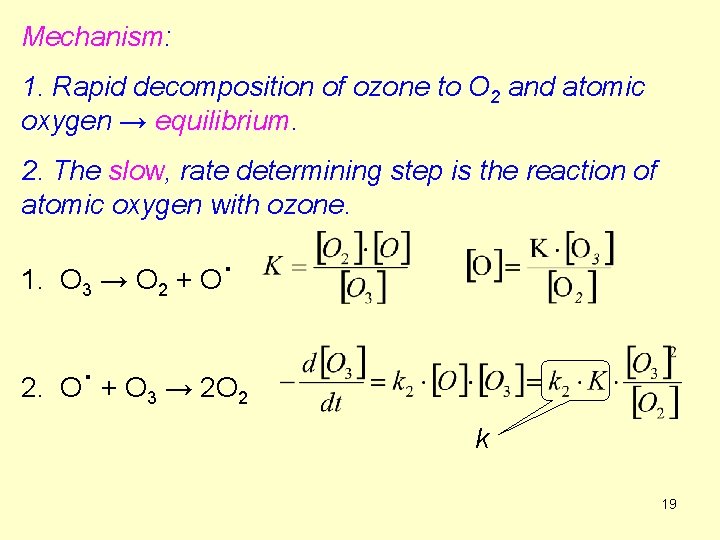
Mechanism: 1. Rapid decomposition of ozone to O 2 and atomic oxygen → equilibrium. 2. The slow, rate determining step is the reaction of atomic oxygen with ozone. 1. O 3 → O 2 2. . O +O 3 . +O → 2 O 2 k 19
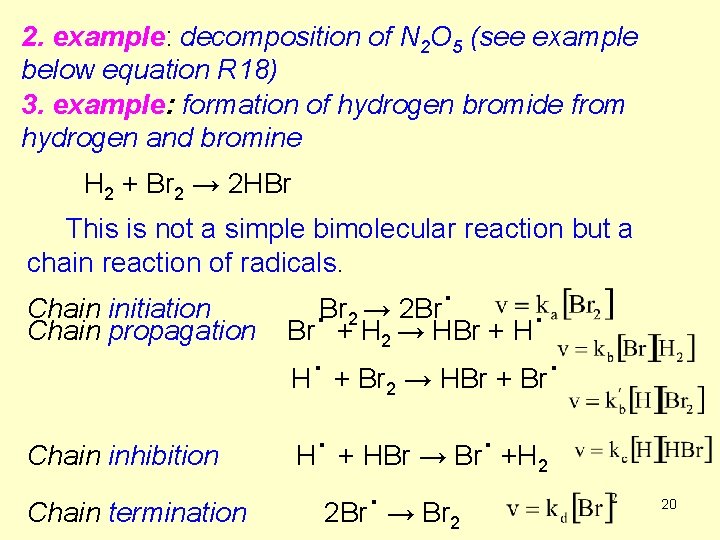
2. example: decomposition of N 2 O 5 (see example below equation R 18) 3. example: formation of hydrogen bromide from hydrogen and bromine H 2 + Br 2 → 2 HBr This is not a simple bimolecular reaction but a chain reaction of radicals. Chain initiation Chain propagation . → 2 Br Br 2. . Br + H 2 → HBr + H. . H + Br → HBr + Br 2 Chain inhibition Chain termination . . H + HBr → Br +H 2. 2 Br → Br 2 20
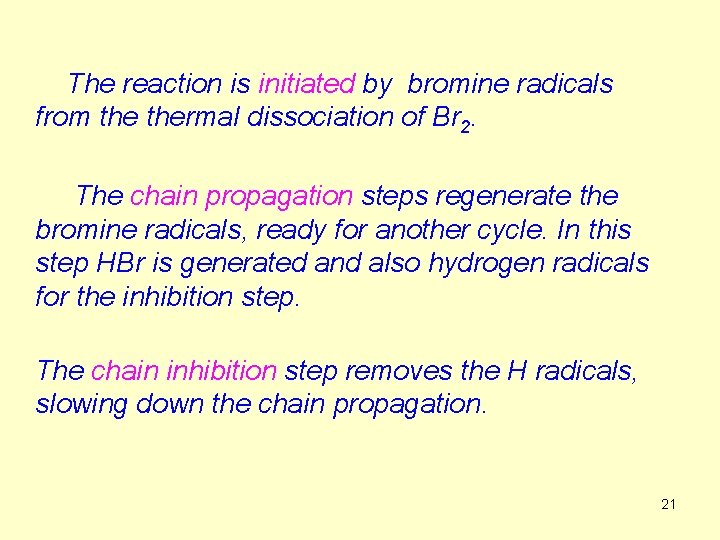
The reaction is initiated by bromine radicals from thermal dissociation of Br 2. The chain propagation steps regenerate the bromine radicals, ready for another cycle. In this step HBr is generated and also hydrogen radicals for the inhibition step. The chain inhibition step removes the H radicals, slowing down the chain propagation. 21
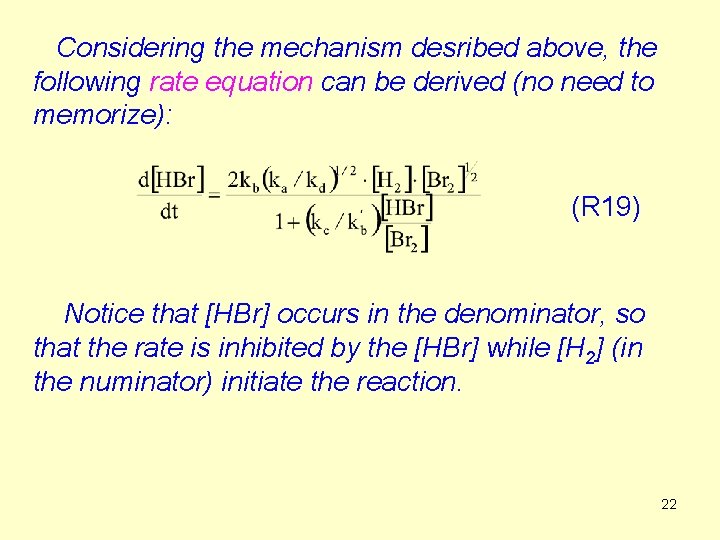
Considering the mechanism desribed above, the following rate equation can be derived (no need to memorize): (R 19) Notice that [HBr] occurs in the denominator, so that the rate is inhibited by the [HBr] while [H 2] (in the numinator) initiate the reaction. 22
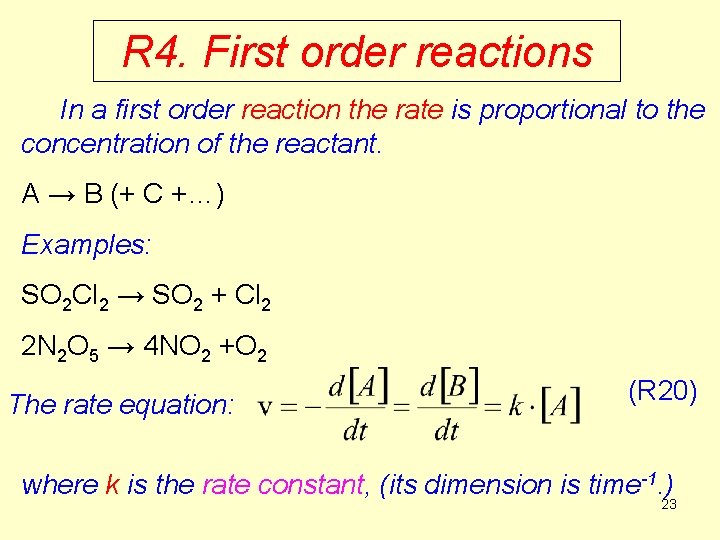
R 4. First order reactions In a first order reaction the rate is proportional to the concentration of the reactant. A → B (+ C +…) Examples: SO 2 Cl 2 → SO 2 + Cl 2 2 N 2 O 5 → 4 NO 2 +O 2 The rate equation: (R 20) where k is the rate constant, (its dimension is time-1. ) 23
![Separate the variables and integrate from t 0 conc A 0 to Separate the variables and integrate from t = 0 (conc. = [A 0]) to](https://slidetodoc.com/presentation_image_h/9fcac2c20e98a062fd11eb0e061bde2e/image-24.jpg)
Separate the variables and integrate from t = 0 (conc. = [A 0]) to time t (conc. = [A]). (R 21 a) (R 21 b) 24
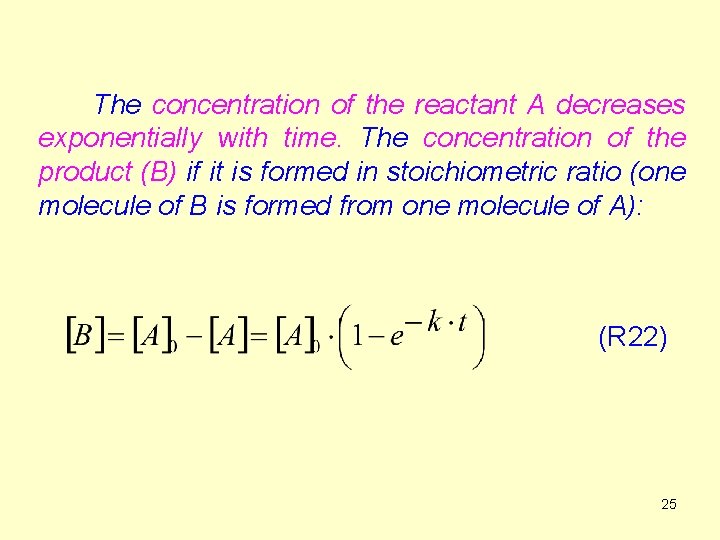
The concentration of the reactant A decreases exponentially with time. The concentration of the product (B) if it is formed in stoichiometric ratio (one molecule of B is formed from one molecule of A): (R 22) 25
![The concentrations as functions of time concentration A0 B A time 26 The concentrations as functions of time. concentration [A]0 [B] [A] time 26](https://slidetodoc.com/presentation_image_h/9fcac2c20e98a062fd11eb0e061bde2e/image-26.jpg)
The concentrations as functions of time. concentration [A]0 [B] [A] time 26
![Linear plot R 21 b lnA0 experimental data a tga k time 27 Linear plot (R 21 b) ln[A]0 experimental data a tga = -k time 27](https://slidetodoc.com/presentation_image_h/9fcac2c20e98a062fd11eb0e061bde2e/image-27.jpg)
Linear plot (R 21 b) ln[A]0 experimental data a tga = -k time 27
![If we plot lnA against time and the data points lie on a staight If we plot ln[A] against time and the data points lie on a staight](https://slidetodoc.com/presentation_image_h/9fcac2c20e98a062fd11eb0e061bde2e/image-28.jpg)
If we plot ln[A] against time and the data points lie on a staight line (within experimental errors), the reaction is of first order. The slope of the line gives the rate constant. Half life t is the time required to reduce the concentration of reactant to half its initial value. (R 23) In case of first order reaction the half life is independent of initial concentration. 28
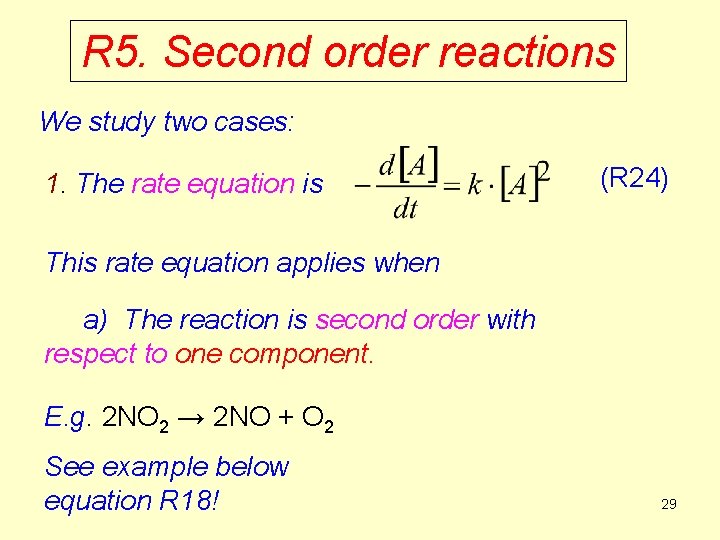
R 5. Second order reactions We study two cases: 1. The rate equation is (R 24) This rate equation applies when a) The reaction is second order with respect to one component. E. g. 2 NO 2 → 2 NO + O 2 See example below equation R 18! 29
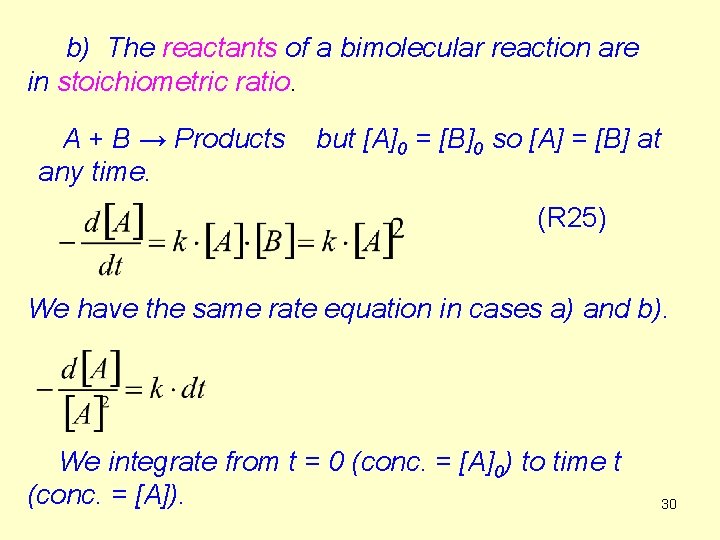
b) The reactants of a bimolecular reaction are in stoichiometric ratio. A + B → Products but [A]0 = [B]0 so [A] = [B] at any time. (R 25) We have the same rate equation in cases a) and b). We integrate from t = 0 (conc. = [A]0) to time t (conc. = [A]). 30
![The integral of 1A2 is 1A const The integrated form of the rate (The integral of 1/[A]2 is -1/[A] +const. ) The integrated form of the rate](https://slidetodoc.com/presentation_image_h/9fcac2c20e98a062fd11eb0e061bde2e/image-31.jpg)
(The integral of 1/[A]2 is -1/[A] +const. ) The integrated form of the rate equation is (R 26) If we plot the reciprocal of the concentration of the reactant against time, we obtain a straight line in case of a second order reaction. The slope is the rate 31 constant.
![Linear plot R 26 1A experimental data a tga k 1A0 time 32 Linear plot (R 26) 1/[A] experimental data a tga = k 1/[A]0 time 32](https://slidetodoc.com/presentation_image_h/9fcac2c20e98a062fd11eb0e061bde2e/image-32.jpg)
Linear plot (R 26) 1/[A] experimental data a tga = k 1/[A]0 time 32
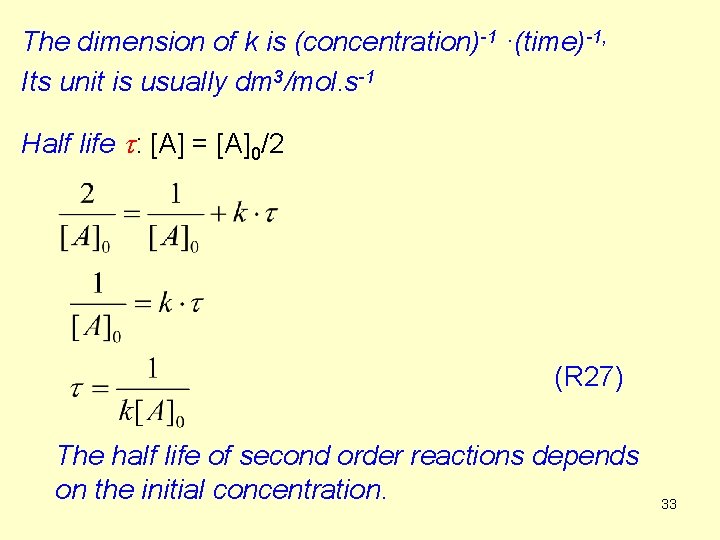
The dimension of k is (concentration)-1 ·(time)-1, Its unit is usually dm 3/mol. s-1 Half life t: [A] = [A]0/2 (R 27) The half life of second order reactions depends on the initial concentration. 33
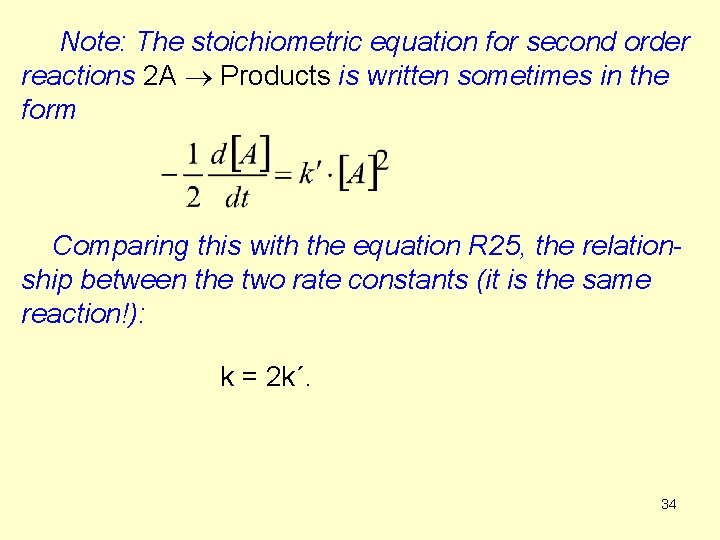
Note: The stoichiometric equation for second order reactions 2 A Products is written sometimes in the form Comparing this with the equation R 25, the relationship between the two rate constants (it is the same reaction!): k = 2 k´. 34
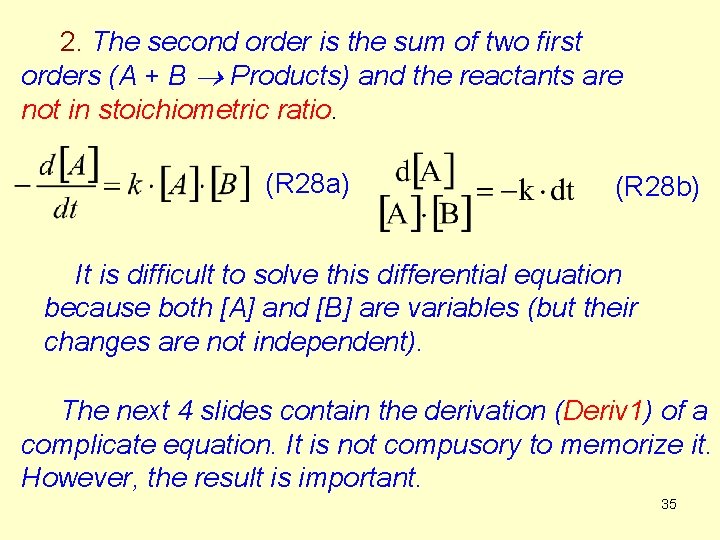
2. The second order is the sum of two first orders (A + B Products) and the reactants are not in stoichiometric ratio. (R 28 a) (R 28 b) It is difficult to solve this differential equation because both [A] and [B] are variables (but their changes are not independent). The next 4 slides contain the derivation (Deriv 1) of a complicate equation. It is not compusory to memorize it. However, the result is important. 35
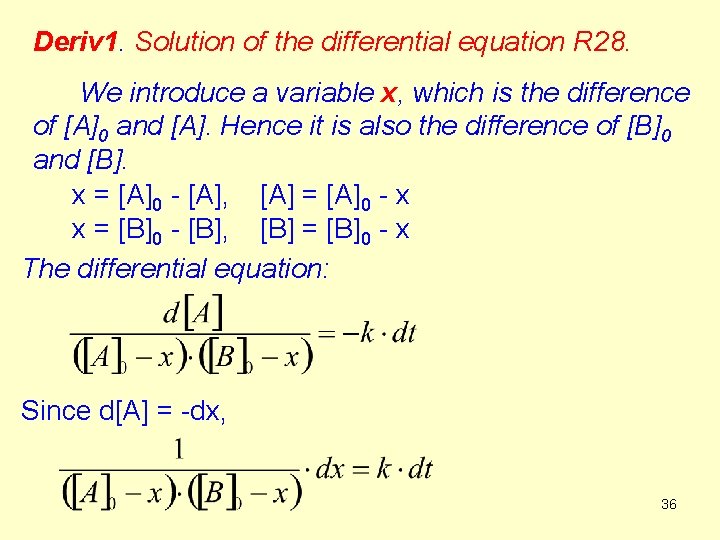
Deriv 1. Solution of the differential equation R 28. We introduce a variable x, which is the difference of [A]0 and [A]. Hence it is also the difference of [B]0 and [B]. x = [A]0 - [A], [A] = [A]0 - x x = [B]0 - [B], [B] = [B]0 - x The differential equation: Since d[A] = -dx, 36
![To alter the left hand side consider the following identity If we substitute A0 To alter the left hand side consider the following identity: If we substitute [A]0](https://slidetodoc.com/presentation_image_h/9fcac2c20e98a062fd11eb0e061bde2e/image-37.jpg)
To alter the left hand side consider the following identity: If we substitute [A]0 -x for a and [B]0 -x for b, the differential equation takes the form 37
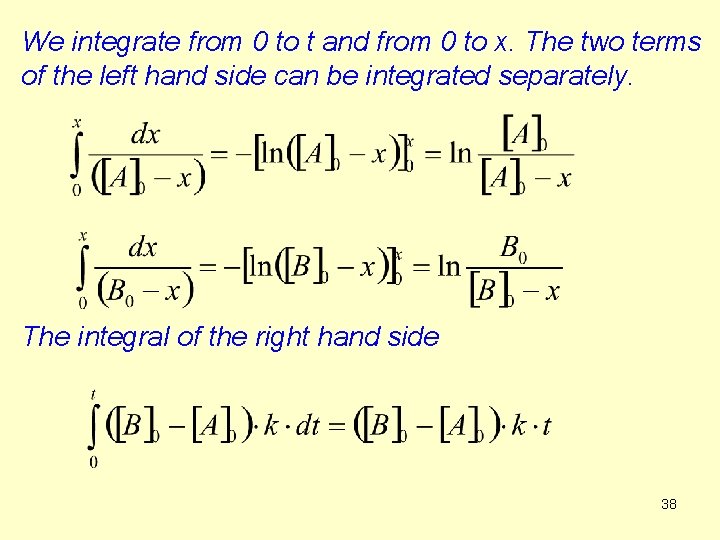
We integrate from 0 to t and from 0 to x. The two terms of the left hand side can be integrated separately. The integral of the right hand side 38
![So the solution of the differential equation Rearrange this equation considering that A0 x So the solution of the differential equation Rearrange this equation considering that [A]0 -x](https://slidetodoc.com/presentation_image_h/9fcac2c20e98a062fd11eb0e061bde2e/image-39.jpg)
So the solution of the differential equation Rearrange this equation considering that [A]0 -x =[A] and [B]0 -x =[B] If we multiply both the first and the second factor of the left hand side by -1, we get to the final form. 39
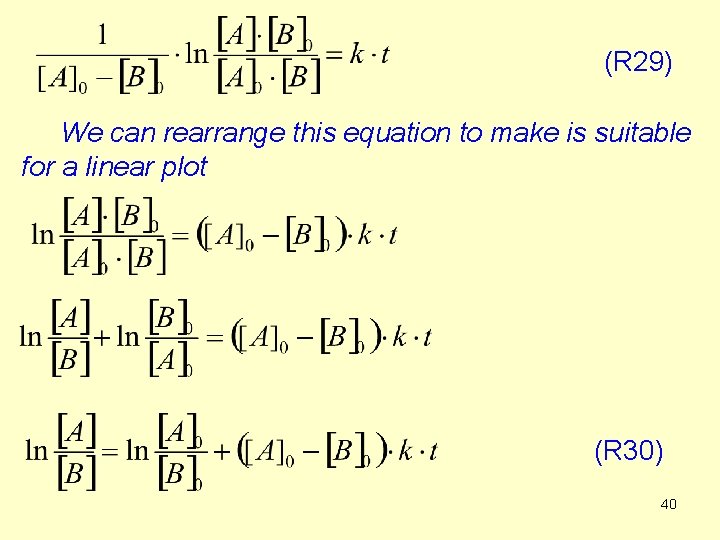
(R 29) We can rearrange this equation to make is suitable for a linear plot (R 30) 40
![Plot lnAB as a function of time experimental data a time 41 Plot ln([A]/[B]) as a function of time experimental data a time 41](https://slidetodoc.com/presentation_image_h/9fcac2c20e98a062fd11eb0e061bde2e/image-41.jpg)
Plot ln([A]/[B]) as a function of time experimental data a time 41
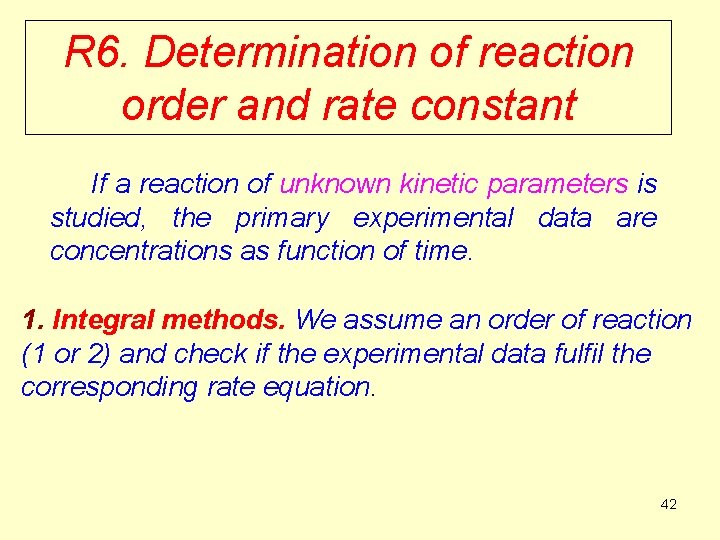
R 6. Determination of reaction order and rate constant If a reaction of unknown kinetic parameters is studied, the primary experimental data are concentrations as function of time. 1. Integral methods. We assume an order of reaction (1 or 2) and check if the experimental data fulfil the corresponding rate equation. 42
![First order R 21 b lnA If the experimental data sit on this like First order (R 21 b) ln[A] If the experimental data sit on this like](https://slidetodoc.com/presentation_image_h/9fcac2c20e98a062fd11eb0e061bde2e/image-43.jpg)
First order (R 21 b) ln[A] If the experimental data sit on this like straight line, the reaction is of first order. time 43
![First order R 21 b lnA If the experimental data do not sit on First order (R 21 b) ln[A] If the experimental data do not sit on](https://slidetodoc.com/presentation_image_h/9fcac2c20e98a062fd11eb0e061bde2e/image-44.jpg)
First order (R 21 b) ln[A] If the experimental data do not sit on the R 21 b like straight line, the reaction is not of first order. time 44
![Second order R 26 1A If the experimental data sit on a this kind Second order (R 26) 1/[A] If the experimental data sit on a this kind](https://slidetodoc.com/presentation_image_h/9fcac2c20e98a062fd11eb0e061bde2e/image-45.jpg)
Second order (R 26) 1/[A] If the experimental data sit on a this kind straight line, the reaction is of second order. time 45
![Second order R 26 1A In this case the experimental data do not sit Second order (R 26) 1/[A] In this case the experimental data do not sit](https://slidetodoc.com/presentation_image_h/9fcac2c20e98a062fd11eb0e061bde2e/image-46.jpg)
Second order (R 26) 1/[A] In this case the experimental data do not sit on a straight line, the reaction is not of second order. time 46
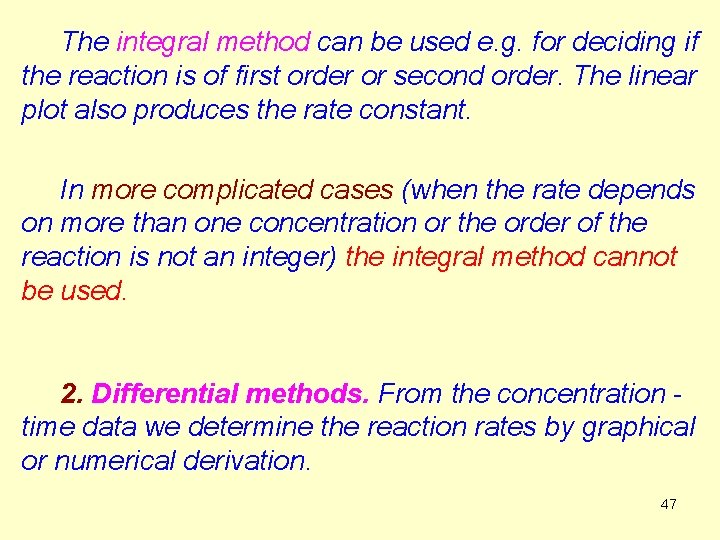
The integral method can be used e. g. for deciding if the reaction is of first order or second order. The linear plot also produces the rate constant. In more complicated cases (when the rate depends on more than one concentration or the order of the reaction is not an integer) the integral method cannot be used. 2. Differential methods. From the concentration - time data we determine the reaction rates by graphical or numerical derivation. 47
![The concentration of a reactant as a function of time concentration A0 R 31 The concentration of a reactant as a function of time. concentration [A]0 (R 31)](https://slidetodoc.com/presentation_image_h/9fcac2c20e98a062fd11eb0e061bde2e/image-48.jpg)
The concentration of a reactant as a function of time. concentration [A]0 (R 31) [A] a t time 48
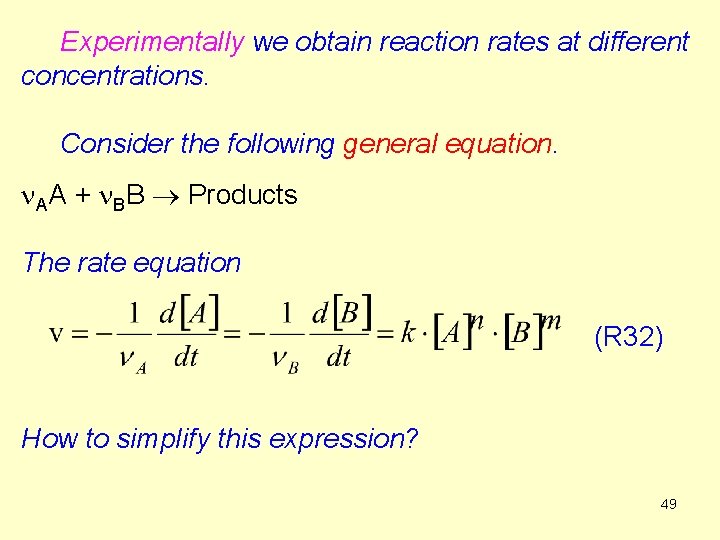
Experimentally we obtain reaction rates at different concentrations. Consider the following general equation. n. AA + n. BB Products The rate equation (R 32) How to simplify this expression? 49
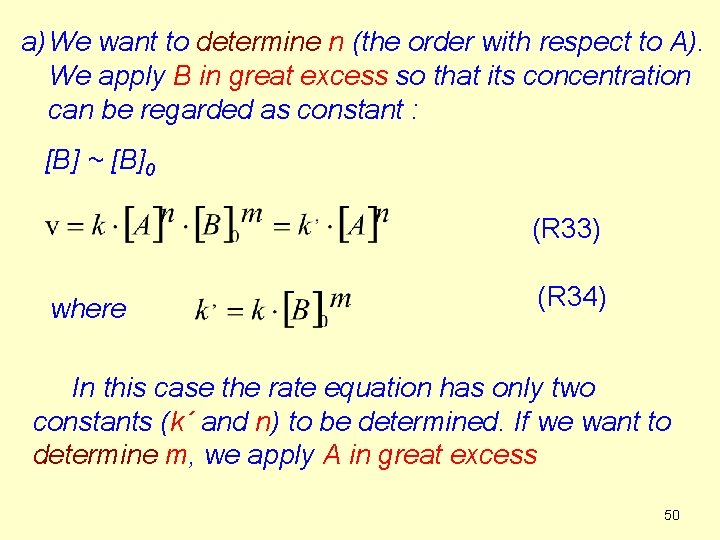
a) We want to determine n (the order with respect to A). We apply B in great excess so that its concentration can be regarded as constant : [B] ~ [B]0 (R 33) where (R 34) In this case the rate equation has only two constants (k´ and n) to be determined. If we want to determine m, we apply A in great excess 50
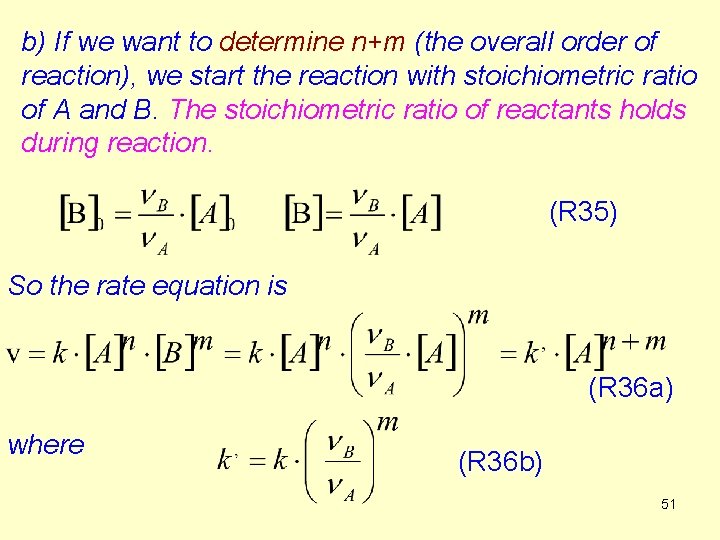
b) If we want to determine n+m (the overall order of reaction), we start the reaction with stoichiometric ratio of A and B. The stoichiometric ratio of reactants holds during reaction. (R 35) So the rate equation is (R 36 a) where (R 36 b) 51
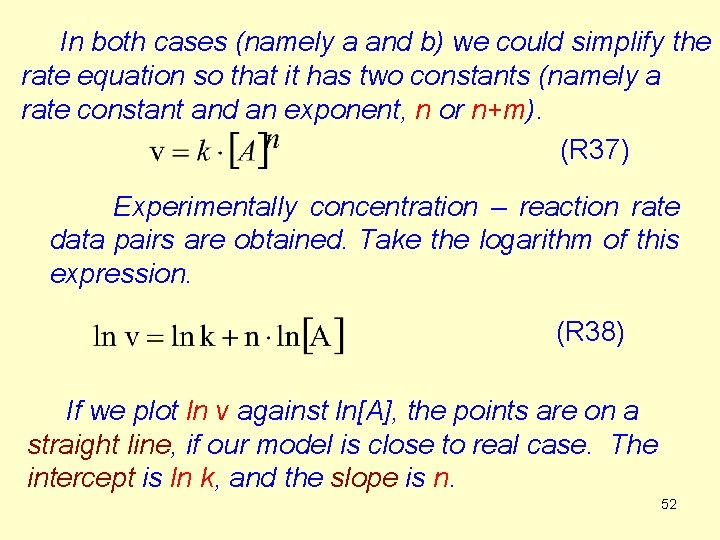
In both cases (namely a and b) we could simplify the rate equation so that it has two constants (namely a rate constant and an exponent, n or n+m). (R 37) Experimentally concentration – reaction rate data pairs are obtained. Take the logarithm of this expression. (R 38) If we plot ln v against ln[A], the points are on a straight line, if our model is close to real case. The intercept is ln k, and the slope is n. 52
![lnv as a function of lnA ln v experimental data a ln k lnA lnv as a function of ln[A] ln v experimental data a ln k ln[A]](https://slidetodoc.com/presentation_image_h/9fcac2c20e98a062fd11eb0e061bde2e/image-53.jpg)
lnv as a function of ln[A] ln v experimental data a ln k ln[A] 53
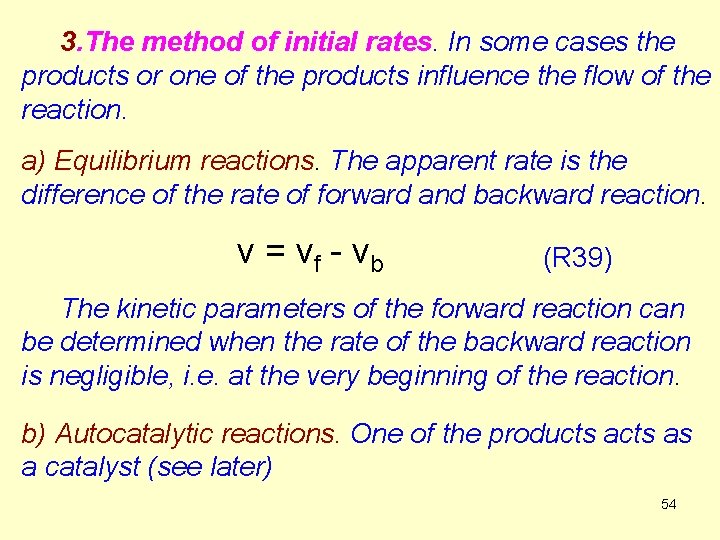
3. The method of initial rates. In some cases the products or one of the products influence the flow of the reaction. a) Equilibrium reactions. The apparent rate is the difference of the rate of forward and backward reaction. v = vf - vb (R 39) The kinetic parameters of the forward reaction can be determined when the rate of the backward reaction is negligible, i. e. at the very beginning of the reaction. b) Autocatalytic reactions. One of the products as a catalyst (see later) 54
![The concentration of a reactant as a function of time A0 a time 55 The concentration of a reactant as a function of time. [A]0 a time 55](https://slidetodoc.com/presentation_image_h/9fcac2c20e98a062fd11eb0e061bde2e/image-55.jpg)
The concentration of a reactant as a function of time. [A]0 a time 55
![The initial rates are determined at various A0 concentrations The kinetic parameters are calculated The initial rates are determined at various [A]0 concentrations. The kinetic parameters are calculated](https://slidetodoc.com/presentation_image_h/9fcac2c20e98a062fd11eb0e061bde2e/image-56.jpg)
The initial rates are determined at various [A]0 concentrations. The kinetic parameters are calculated from [A]0 – v 0 data pairs. If the form of the kinetic equation is (R 40 a) it is valid for the initial rates, too: (R 40 b) We change the initial contentration Ao and plot ln v 0 against ln[A]0, the points are on a straight line. The intercept is lnk, and the slope is n. The initial reaction velocity is determined measuring the concentration during the initial part of the reaction. 56
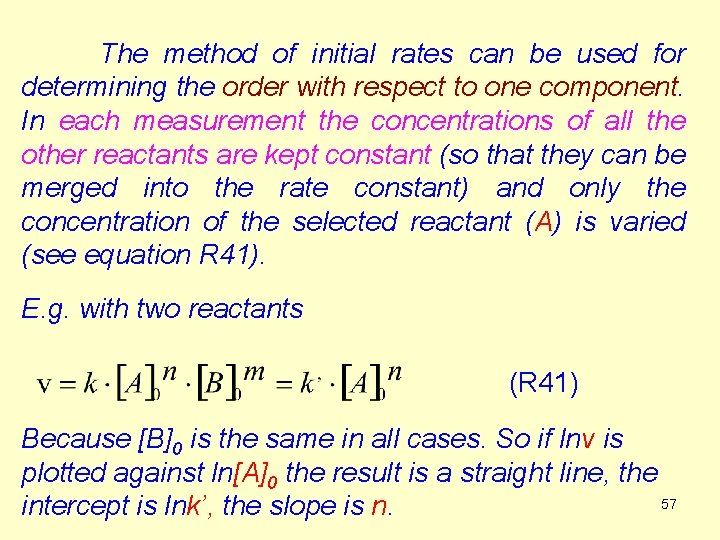
The method of initial rates can be used for determining the order with respect to one component. In each measurement the concentrations of all the other reactants are kept constant (so that they can be merged into the rate constant) and only the concentration of the selected reactant (A) is varied (see equation R 41). E. g. with two reactants (R 41) Because [B]0 is the same in all cases. So if lnv is plotted against ln[A]0 the result is a straight line, the 57 intercept is lnk’, the slope is n.
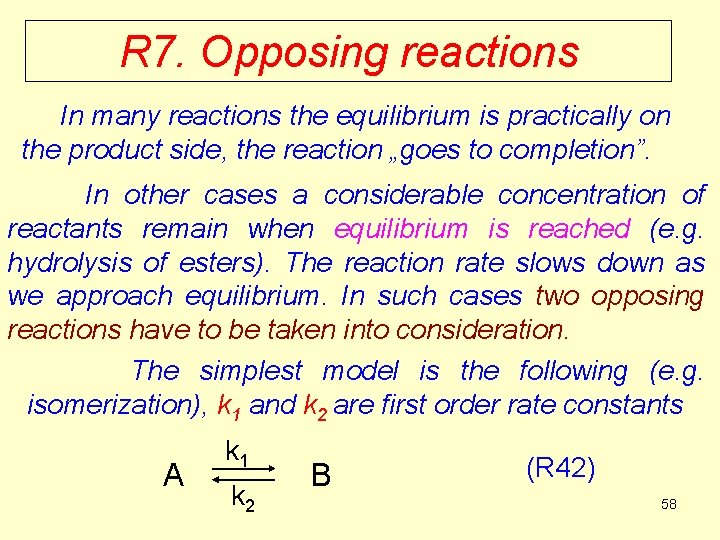
R 7. Opposing reactions In many reactions the equilibrium is practically on the product side, the reaction „goes to completion”. In other cases a considerable concentration of reactants remain when equilibrium is reached (e. g. hydrolysis of esters). The reaction rate slows down as we approach equilibrium. In such cases two opposing reactions have to be taken into consideration. The simplest model is the following (e. g. isomerization), k 1 and k 2 are first order rate constants A k 1 k 2 B (R 42) 58
![The rate equation R 43 At equilibrium v 0 k 1Ae k The rate equation (R 43) At equilibrium v = 0, k 1·[A]e = k](https://slidetodoc.com/presentation_image_h/9fcac2c20e98a062fd11eb0e061bde2e/image-59.jpg)
The rate equation (R 43) At equilibrium v = 0, k 1·[A]e = k 2·[B]e , (R 44) where [A]e and [B]e are the equilibrium concentrations of A and B, respectively. The equilibrium constant in terms of concentrations: (R 45) So the equilibrium constant is equal to the ratio of rate constants of the forward (k 1) and backward (k 2) 59 reactions, respectively.
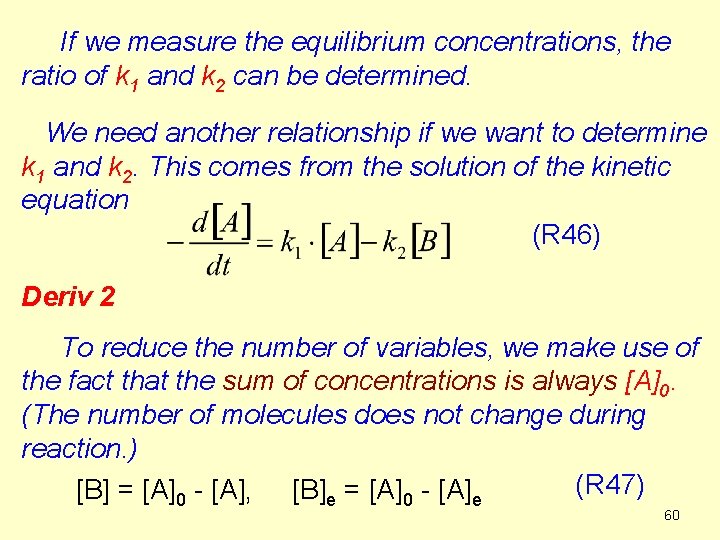
If we measure the equilibrium concentrations, the ratio of k 1 and k 2 can be determined. We need another relationship if we want to determine k 1 and k 2. This comes from the solution of the kinetic equation (R 46) Deriv 2 To reduce the number of variables, we make use of the fact that the sum of concentrations is always [A]0. (The number of molecules does not change during reaction. ) (R 47) [B] = [A]0 - [A], [B]e = [A]0 - [A]e 60
![Before integration we change the last term k 2A0 61 Before integration we change the last term (k 2·[A]0). 61](https://slidetodoc.com/presentation_image_h/9fcac2c20e98a062fd11eb0e061bde2e/image-61.jpg)
Before integration we change the last term (k 2·[A]0). 61
![We integrate this differential equation from A0 to A and from 0 to t We integrate this differential equation from [A]0 to [A] and from 0 to t](https://slidetodoc.com/presentation_image_h/9fcac2c20e98a062fd11eb0e061bde2e/image-62.jpg)
We integrate this differential equation from [A]0 to [A] and from 0 to t 62
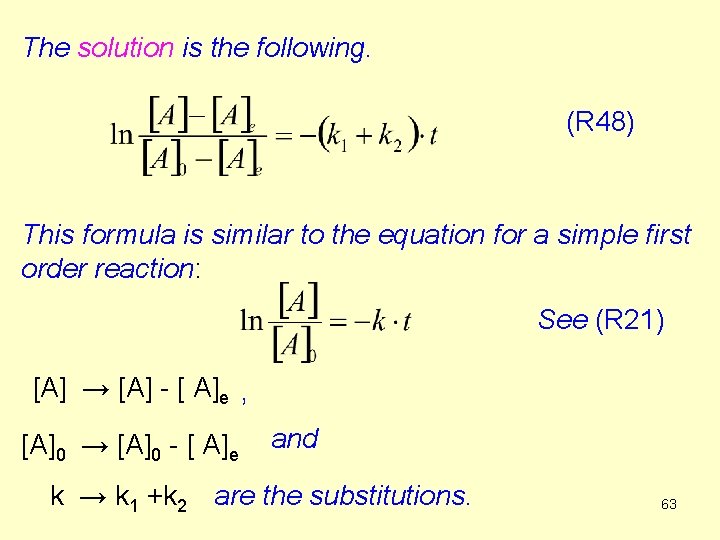
The solution is the following. (R 48) This formula is similar to the equation for a simple first order reaction: See (R 21) [A] → [A] - [ A]e , [A]0 → [A]0 - [ A]e and k → k 1 +k 2 are the substitutions. 63
![We can determine k 1k 2 if we plot lnAA0 against time lnAAe experimental We can determine k 1+k 2 if we plot ln([A]-[A]0) against time. ln([A]-[A]e) experimental](https://slidetodoc.com/presentation_image_h/9fcac2c20e98a062fd11eb0e061bde2e/image-64.jpg)
We can determine k 1+k 2 if we plot ln([A]-[A]0) against time. ln([A]-[A]e) experimental data ln([A]0 -[A]e) tga = -(k 1+k 2) a time 64
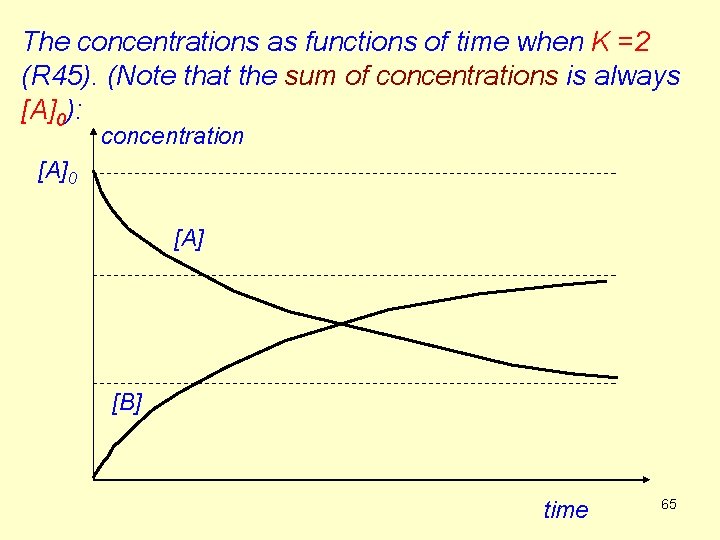
The concentrations as functions of time when K =2 (R 45). (Note that the sum of concentrations is always [A]0): concentration [A]0 [A] [B] time 65
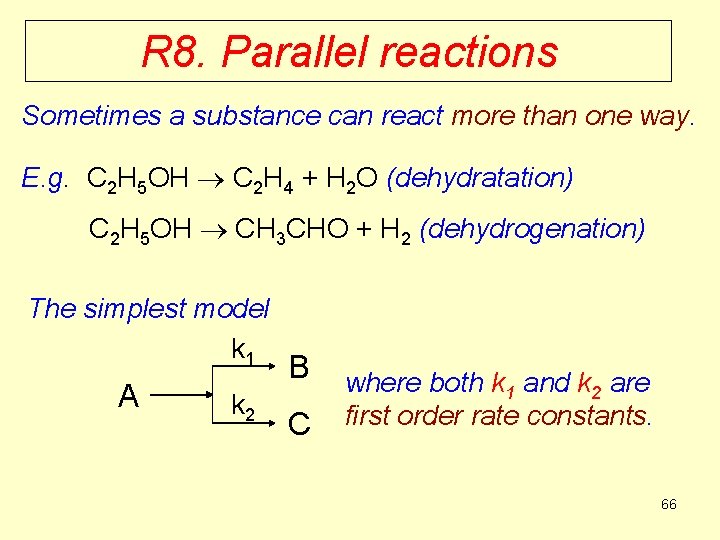
R 8. Parallel reactions Sometimes a substance can react more than one way. E. g. C 2 H 5 OH C 2 H 4 + H 2 O (dehydratation) C 2 H 5 OH CH 3 CHO + H 2 (dehydrogenation) The simplest model k 1 A k 2 B C where both k 1 and k 2 are first order rate constants. 66
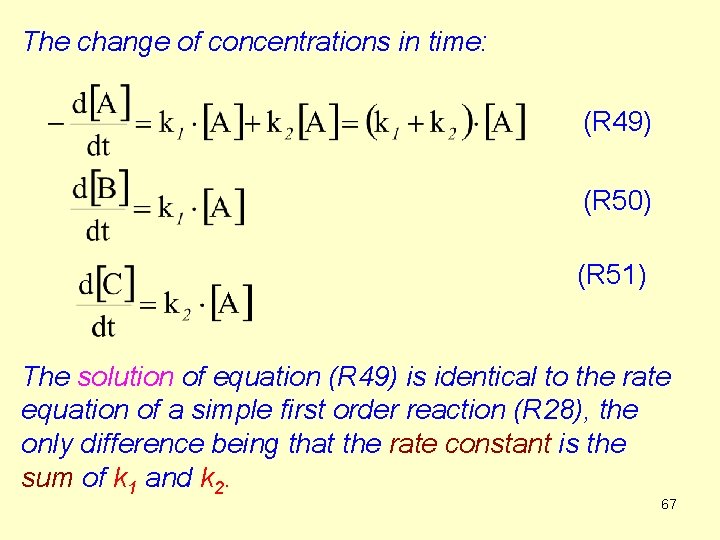
The change of concentrations in time: (R 49) (R 50) (R 51) The solution of equation (R 49) is identical to the rate equation of a simple first order reaction (R 28), the only difference being that the rate constant is the sum of k 1 and k 2. 67
![Time dependence of the concentration of A R 52 lnA is a linear Time dependence of the concentration of A (R 52) ln[A] is a linear](https://slidetodoc.com/presentation_image_h/9fcac2c20e98a062fd11eb0e061bde2e/image-68.jpg)
Time dependence of the concentration of A (R 52) ln[A] is a linear function of time. (k 1+k 2) can be determined from the slope. 68
![Linear plot lnA experimental data lnA0 tga k 1k 2 a time 69 Linear plot ln[A] experimental data ln[A]0 tga = -(k 1+k 2) a time 69](https://slidetodoc.com/presentation_image_h/9fcac2c20e98a062fd11eb0e061bde2e/image-69.jpg)
Linear plot ln[A] experimental data ln[A]0 tga = -(k 1+k 2) a time 69
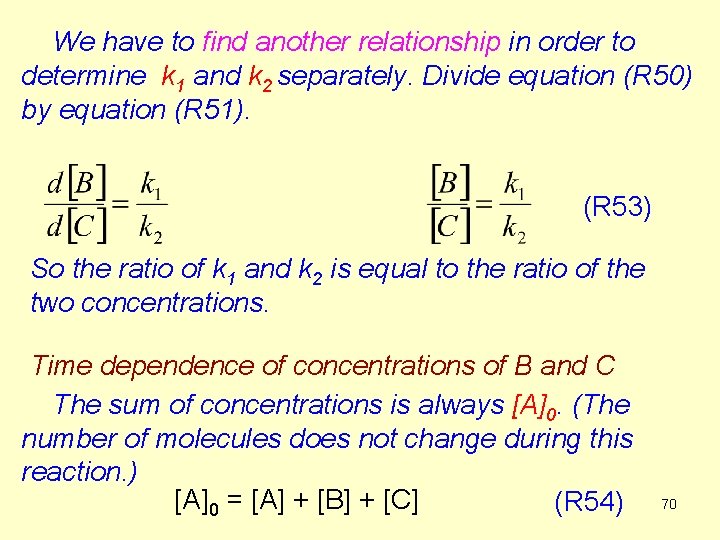
We have to find another relationship in order to determine k 1 and k 2 separately. Divide equation (R 50) by equation (R 51). (R 53) So the ratio of k 1 and k 2 is equal to the ratio of the two concentrations. Time dependence of concentrations of B and C The sum of concentrations is always [A]0. (The number of molecules does not change during this reaction. ) [A]0 = [A] + [B] + [C] (R 54) 70
![Using equation R 52 If we want the time dependence of B eliminate C Using equation (R 52) If we want the time dependence of [B], eliminate [C]](https://slidetodoc.com/presentation_image_h/9fcac2c20e98a062fd11eb0e061bde2e/image-71.jpg)
Using equation (R 52) If we want the time dependence of [B], eliminate [C] using equation (R 53). 71
![Results R 55 By similar consideration the time dependence of C R 56 The Results: (R 55) By similar consideration the time dependence of [C] (R 56) The](https://slidetodoc.com/presentation_image_h/9fcac2c20e98a062fd11eb0e061bde2e/image-72.jpg)
Results: (R 55) By similar consideration the time dependence of [C] (R 56) The sum of the three concentrations (see R 52, R 55 and R 56) is equal to the initial concentration of A according to R 54. 72
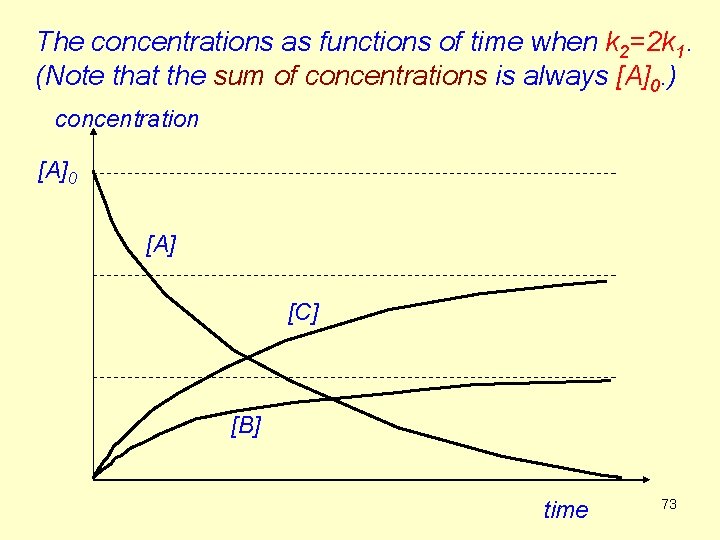
The concentrations as functions of time when k 2=2 k 1. (Note that the sum of concentrations is always [A]0. ) concentration [A]0 [A] [C] [B] time 73
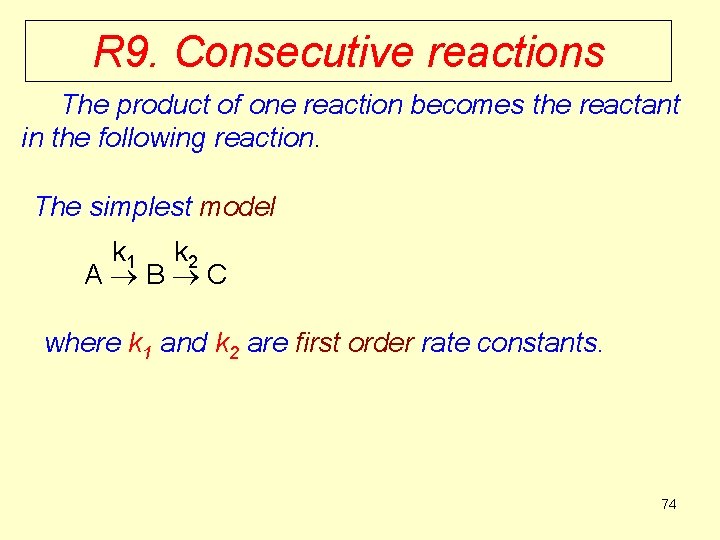
R 9. Consecutive reactions The product of one reaction becomes the reactant in the following reaction. The simplest model k 1 k 2 A B C where k 1 and k 2 are first order rate constants. 74
![Substituting the right hand side of this expression for A into equation R 57 Substituting the right hand side of this expression for [A] into equation R 57](https://slidetodoc.com/presentation_image_h/9fcac2c20e98a062fd11eb0e061bde2e/image-75.jpg)
Substituting the right hand side of this expression for [A] into equation R 57 b we have (R 59) The solution of this differential equation gives the time dependence of [B]. The general solution Rearranging equation R 59 into a standard form: (R 60) 76
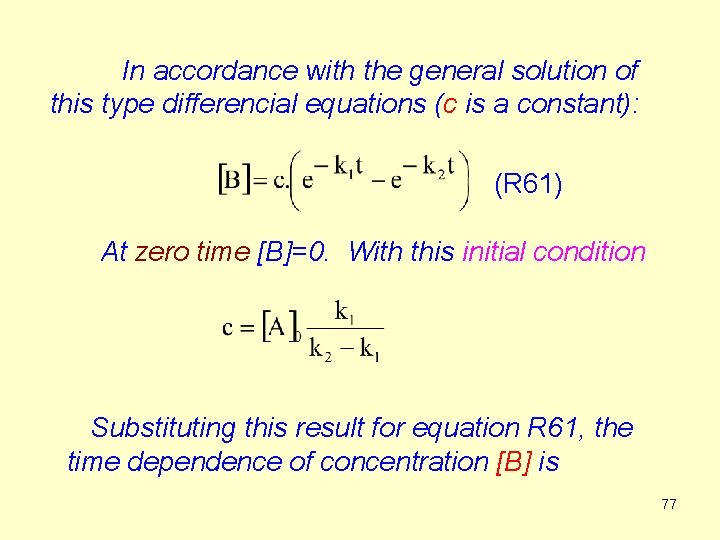
In accordance with the general solution of this type differencial equations (c is a constant): (R 61) At zero time [B]=0. With this initial condition Substituting this result for equation R 61, the time dependence of concentration [B] is 77
![R 62 The concentration of A decreases exponentionally B reaches a maximum then decreases (R 62) The concentration of A decreases exponentionally. [B] reaches a maximum, then decreases](https://slidetodoc.com/presentation_image_h/9fcac2c20e98a062fd11eb0e061bde2e/image-77.jpg)
(R 62) The concentration of A decreases exponentionally. [B] reaches a maximum, then decreases gradually to zero. [C] at first slowly increases, then goes through an inflection and eventually approaches [A]0. Since [C] = [A]0 - [A] - [B] We have (R 63) 78
![The concentrations as functions of time concentration A0 A C B tm time 79 The concentrations as functions of time. concentration [A]0 [A] [C] [B] tm time 79](https://slidetodoc.com/presentation_image_h/9fcac2c20e98a062fd11eb0e061bde2e/image-78.jpg)
The concentrations as functions of time. concentration [A]0 [A] [C] [B] tm time 79
![How to find the maximum of the B t curve when B is How to find the maximum of the [B] – t curve (when [B] is](https://slidetodoc.com/presentation_image_h/9fcac2c20e98a062fd11eb0e061bde2e/image-79.jpg)
How to find the maximum of the [B] – t curve (when [B] is the highests) ? At the maximum the first derivative of the [B] – t curve is zero. where tm is the time at the maximum of [B]. Since the first factor is not zero, the second factor must be zero. 80
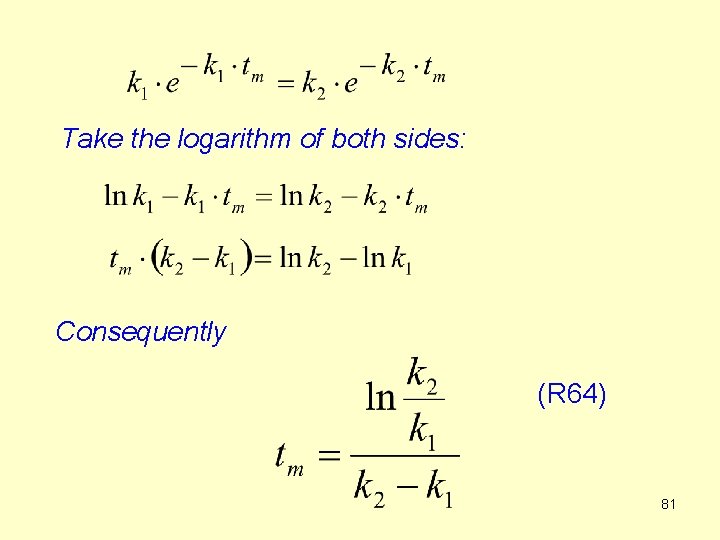
Take the logarithm of both sides: Consequently (R 64) 81
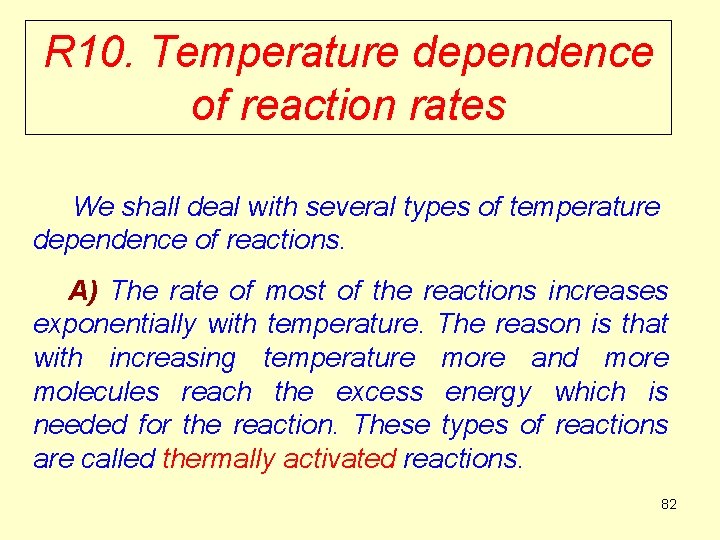
R 10. Temperature dependence of reaction rates We shall deal with several types of temperature dependence of reactions. A) The rate of most of the reactions increases exponentially with temperature. The reason is that with increasing temperature more and more molecules reach the excess energy which is needed for the reaction. These types of reactions are called thermally activated reactions. 82
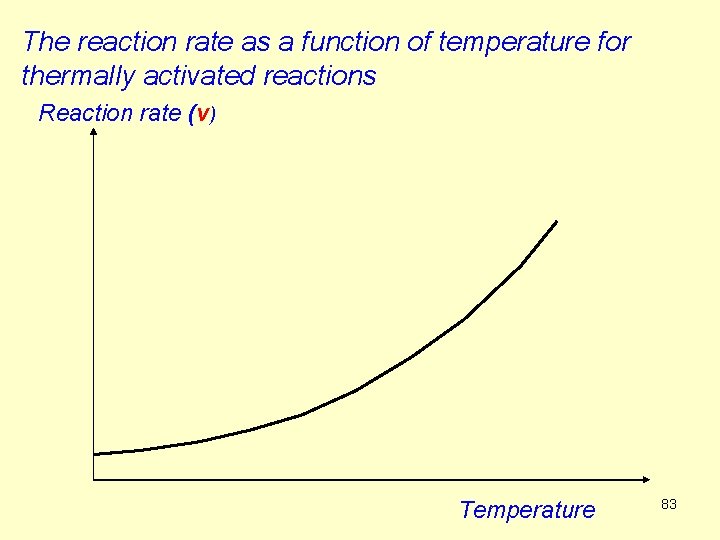
The reaction rate as a function of temperature for thermally activated reactions Reaction rate (v) Temperature 83
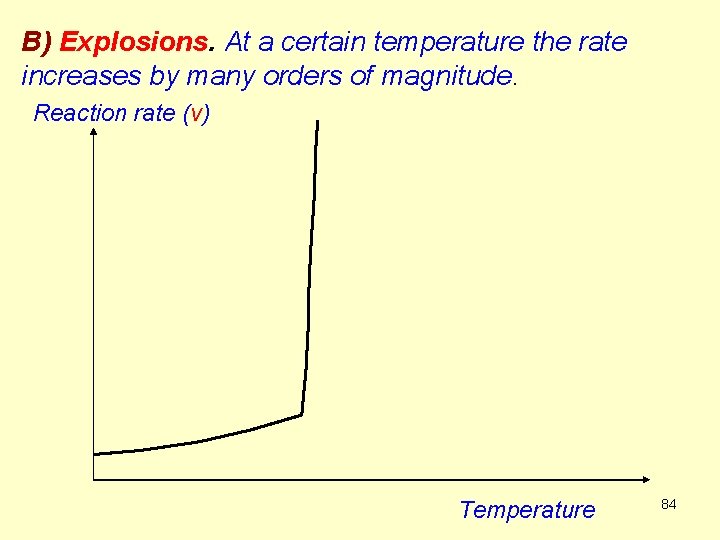
B) Explosions. At a certain temperature the rate increases by many orders of magnitude. Reaction rate (v) Temperature 84
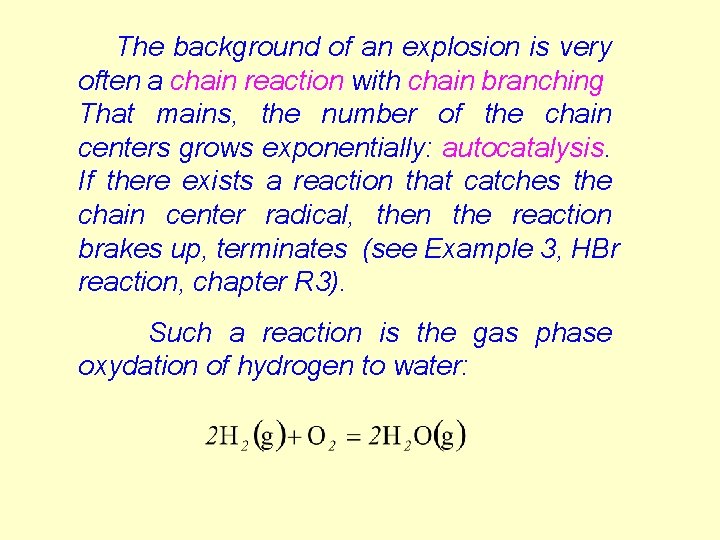
The background of an explosion is very often a chain reaction with chain branching That mains, the number of the chain centers grows exponentially: autocatalysis. If there exists a reaction that catches the chain center radical, then the reaction brakes up, terminates (see Example 3, HBr reaction, chapter R 3). Such a reaction is the gas phase oxydation of hydrogen to water:
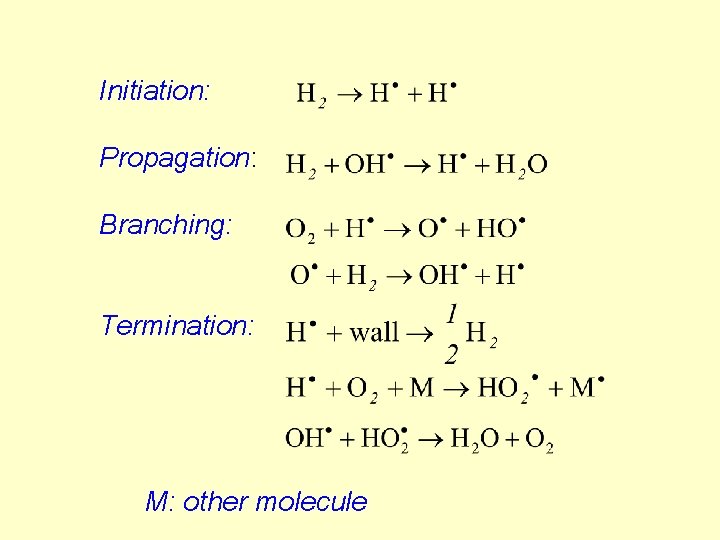
Initiation: Propagation: Branching: Termination: M: other molecule
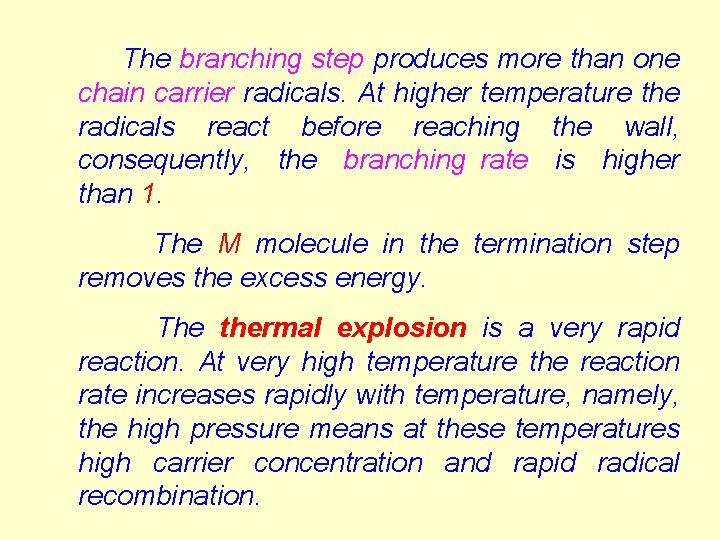
The branching step produces more than one chain carrier radicals. At higher temperature the radicals react before reaching the wall, consequently, the branching rate is higher than 1. The M molecule in the termination step removes the excess energy. The thermal explosion is a very rapid reaction. At very high temperature the reaction rate increases rapidly with temperature, namely, the high pressure means at these temperatures high carrier concentration and rapid radical recombination.
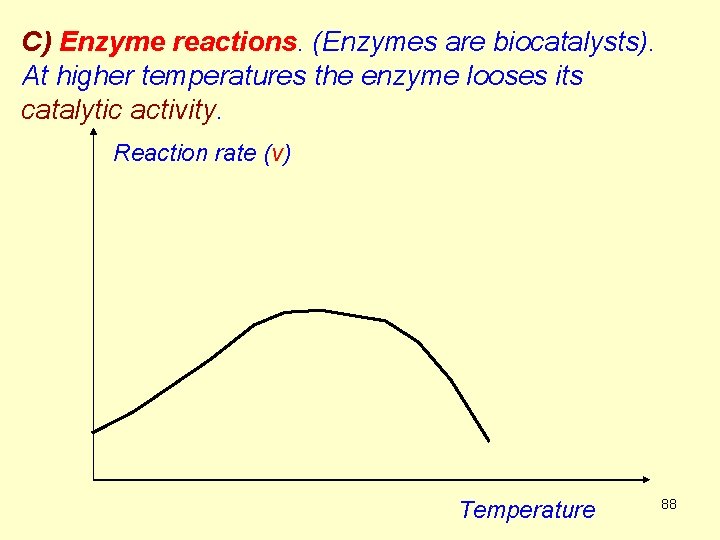
C) Enzyme reactions. (Enzymes are biocatalysts). At higher temperatures the enzyme looses its catalytic activity. Reaction rate (v) Temperature 88
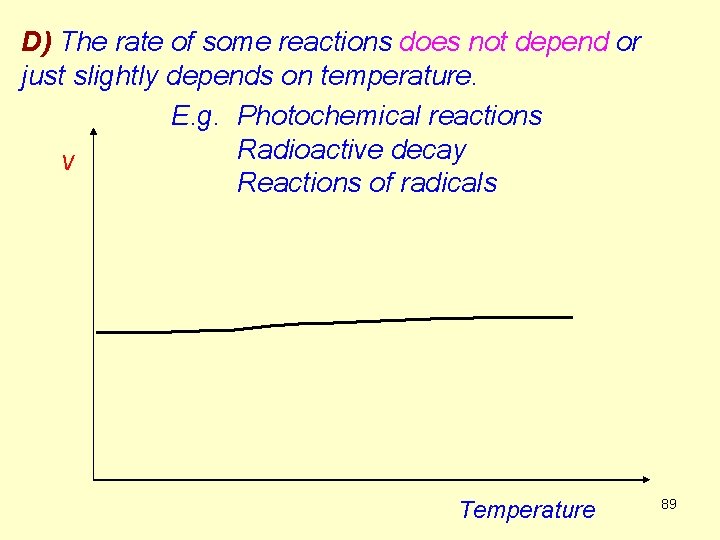
D) The rate of some reactions does not depend or just slightly depends on temperature. E. g. Photochemical reactions Radioactive decay v Reactions of radicals Temperature 89
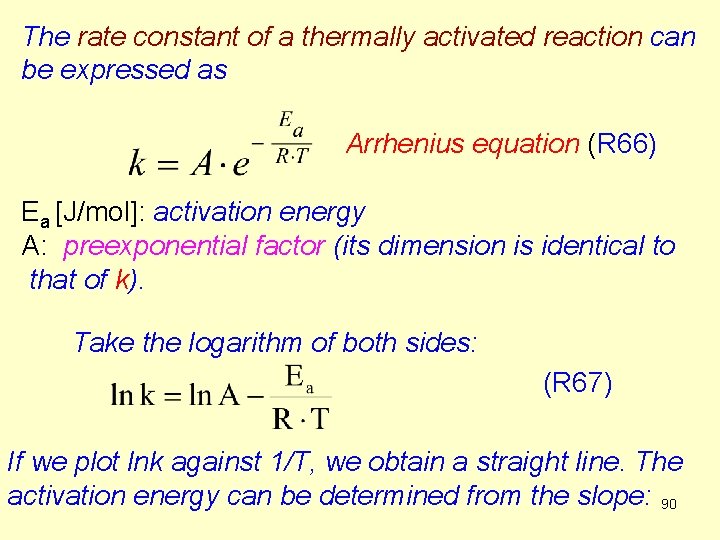
The rate constant of a thermally activated reaction can be expressed as Arrhenius equation (R 66) Ea [J/mol]: activation energy A: preexponential factor (its dimension is identical to that of k). Take the logarithm of both sides: (R 67) If we plot lnk against 1/T, we obtain a straight line. The activation energy can be determined from the slope: 90
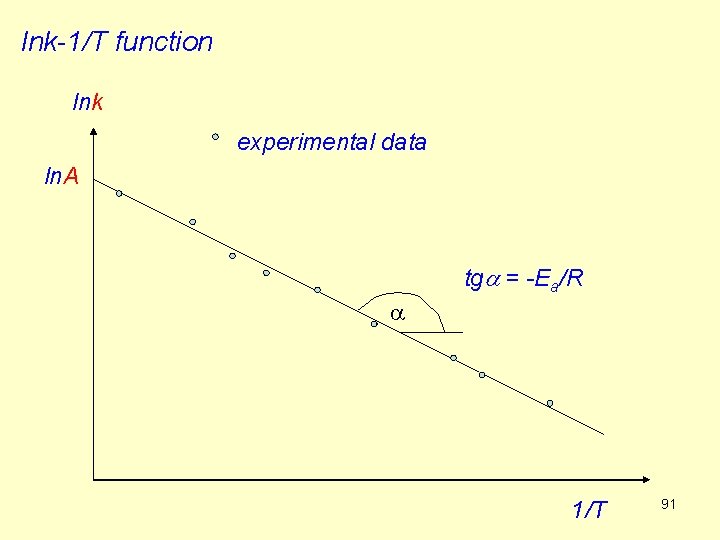
lnk-1/T function lnk experimental data ln. A tga = -Ea/R a 1/T 91
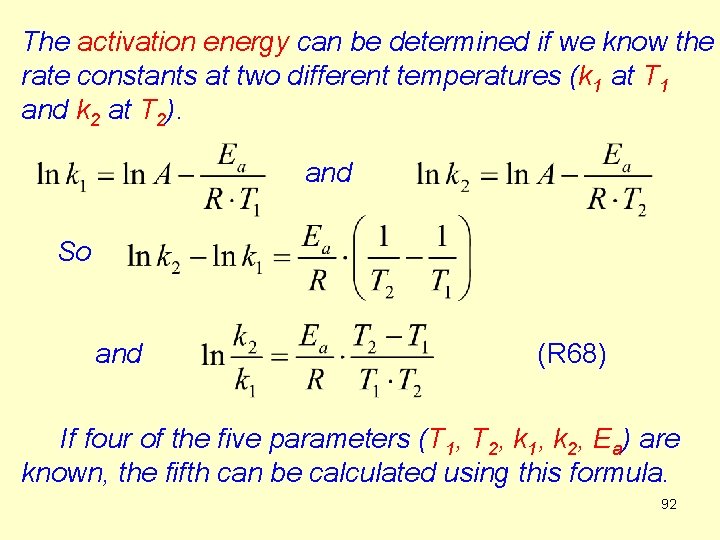
The activation energy can be determined if we know the rate constants at two different temperatures (k 1 at T 1 and k 2 at T 2). and So and (R 68) If four of the five parameters (T 1, T 2, k 1, k 2, Ea) are known, the fifth can be calculated using this formula. 92
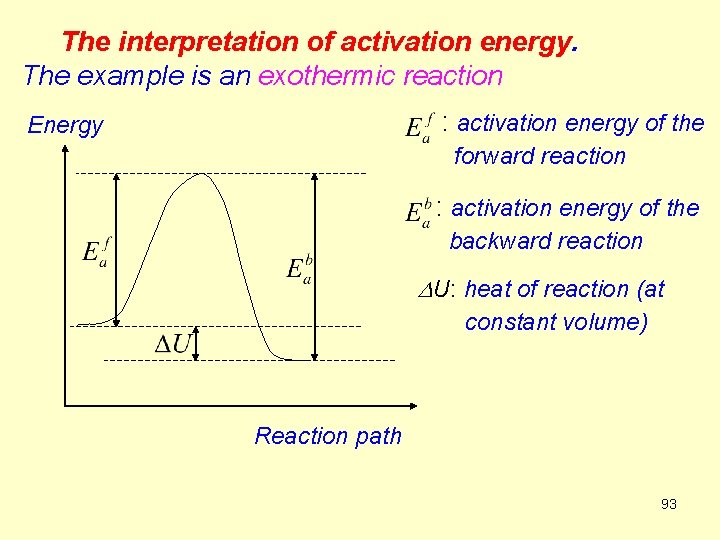
The interpretation of activation energy. The example is an exothermic reaction : activation energy of the Energy forward reaction : activation energy of the backward reaction DU: heat of reaction (at constant volume) Reaction path 93
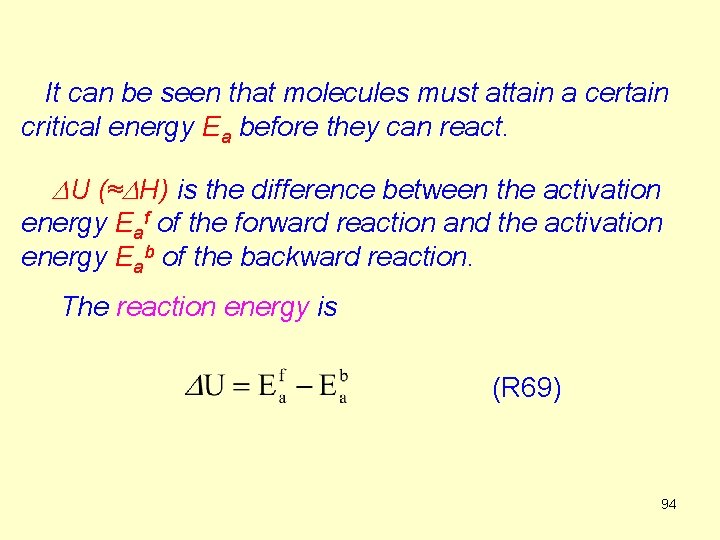
It can be seen that molecules must attain a certain critical energy Ea before they can react. DU (≈DH) is the difference between the activation energy Eaf of the forward reaction and the activation energy Eab of the backward reaction. The reaction energy is (R 69) 94
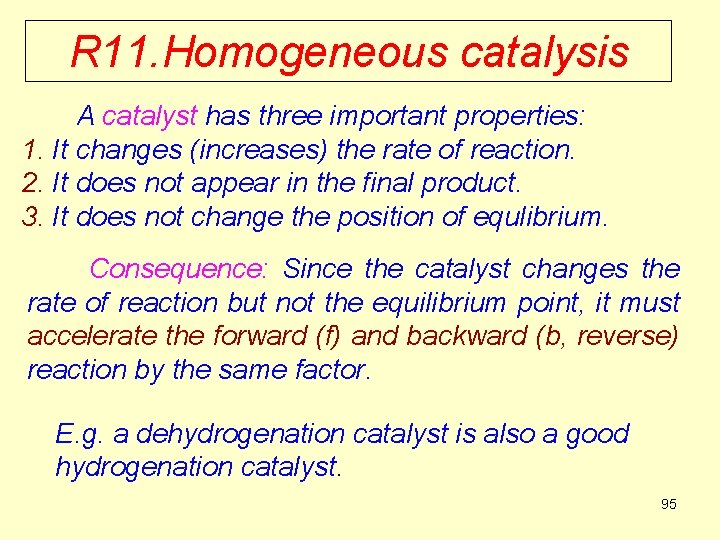
R 11. Homogeneous catalysis A catalyst has three important properties: 1. It changes (increases) the rate of reaction. 2. It does not appear in the final product. 3. It does not change the position of equlibrium. Consequence: Since the catalyst changes the rate of reaction but not the equilibrium point, it must accelerate the forward (f) and backward (b, reverse) reaction by the same factor. E. g. a dehydrogenation catalyst is also a good hydrogenation catalyst. 95
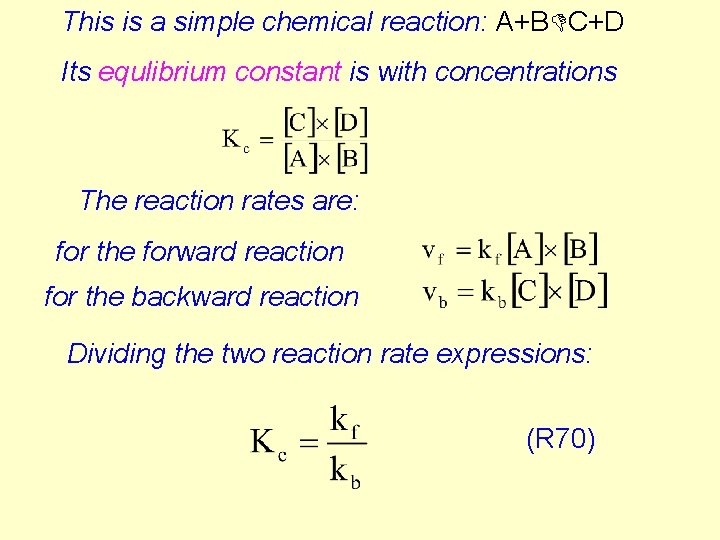
This is a simple chemical reaction: A+BDC+D Its equlibrium constant is with concentrations The reaction rates are: for the forward reaction for the backward reaction Dividing the two reaction rate expressions: (R 70)
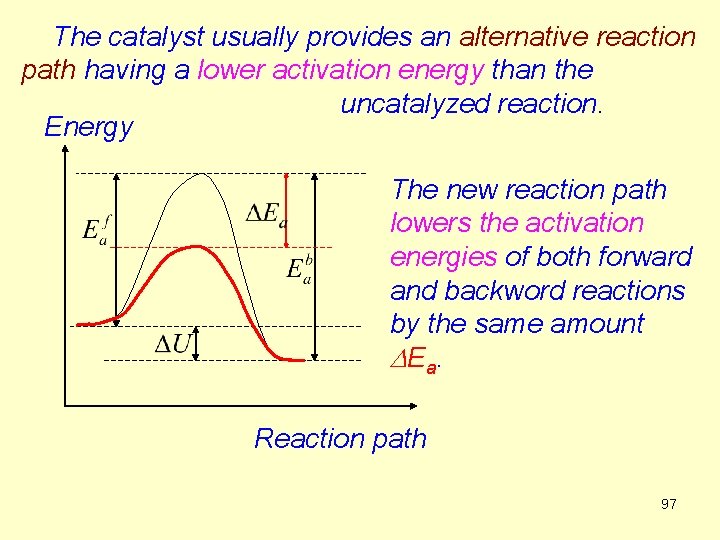
The catalyst usually provides an alternative reaction path having a lower activation energy than the uncatalyzed reaction. Energy The new reaction path lowers the activation energies of both forward and backword reactions by the same amount D E a. Reaction path 97
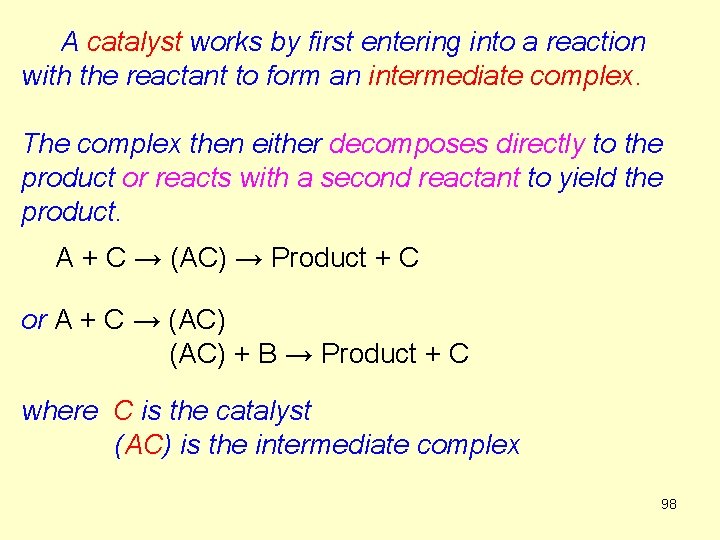
A catalyst works by first entering into a reaction with the reactant to form an intermediate complex. The complex then either decomposes directly to the product or reacts with a second reactant to yield the product. A + C → (AC) → Product + C or A + C → (AC) + B → Product + C where C is the catalyst (AC) is the intermediate complex 98
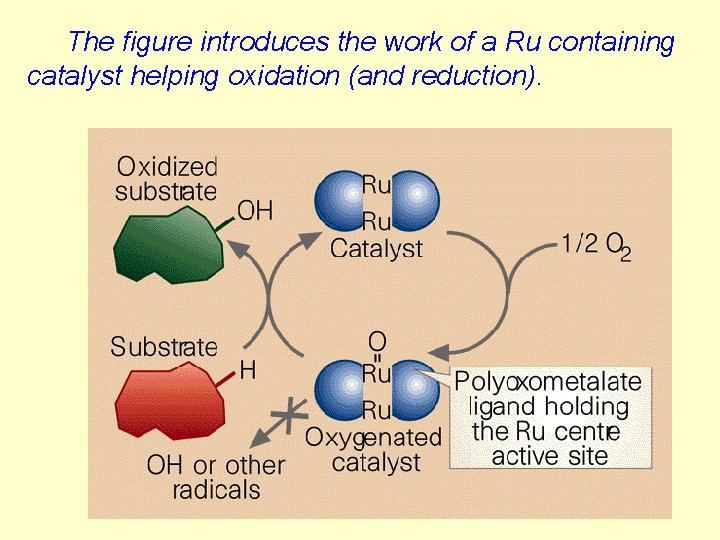
The figure introduces the work of a Ru containing catalyst helping oxidation (and reduction).
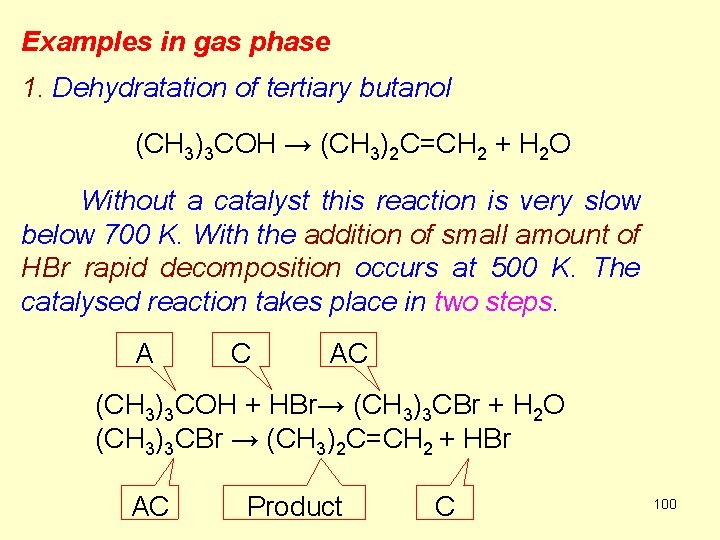
Examples in gas phase 1. Dehydratation of tertiary butanol (CH 3)3 COH → (CH 3)2 C=CH 2 + H 2 O Without a catalyst this reaction is very slow below 700 K. With the addition of small amount of HBr rapid decomposition occurs at 500 K. The catalysed reaction takes place in two steps. A C AC (CH 3)3 COH + HBr→ (CH 3)3 CBr + H 2 O (CH 3)3 CBr → (CH 3)2 C=CH 2 + HBr AC Product C 100
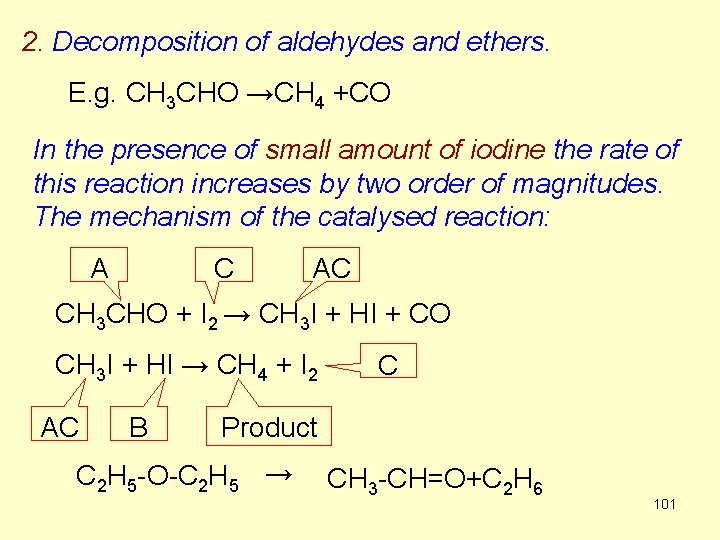
2. Decomposition of aldehydes and ethers. E. g. CH 3 CHO →CH 4 +CO In the presence of small amount of iodine the rate of this reaction increases by two order of magnitudes. The mechanism of the catalysed reaction: A C AC CH 3 CHO + I 2 → CH 3 I + HI + CO CH 3 I + HI → CH 4 + I 2 AC B C Product C 2 H 5 -O-C 2 H 5 → CH 3 -CH=O+C 2 H 6 101
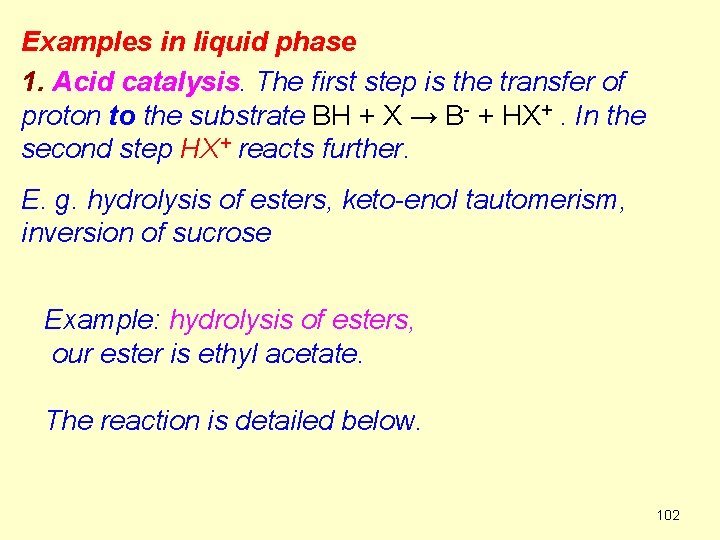
Examples in liquid phase 1. Acid catalysis. The first step is the transfer of proton to the substrate BH + X → B- + HX+. In the second step HX+ reacts further. E. g. hydrolysis of esters, keto-enol tautomerism, inversion of sucrose Example: hydrolysis of esters, our ester is ethyl acetate. The reaction is detailed below. 102
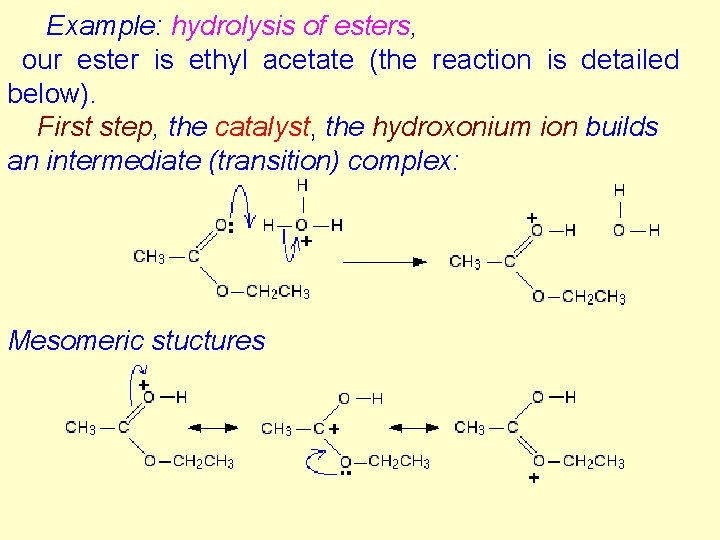
Example: hydrolysis of esters, our ester is ethyl acetate (the reaction is detailed below). First step, the catalyst, the hydroxonium ion builds an intermediate (transition) complex: Mesomeric stuctures
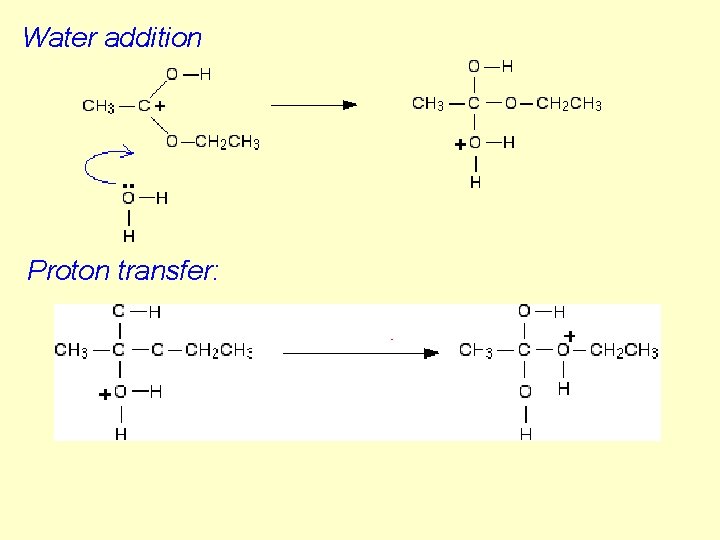
Water addition Proton transfer:
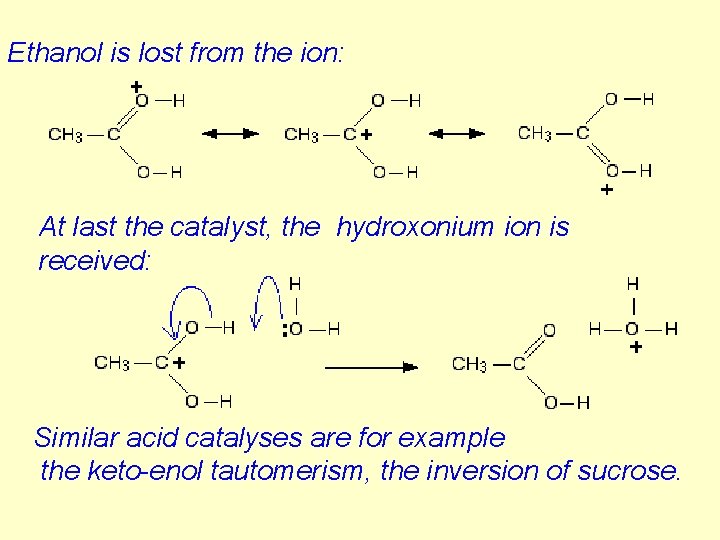
Ethanol is lost from the ion: At last the catalyst, the hydroxonium ion is received: Similar acid catalyses are for example the keto-enol tautomerism, the inversion of sucrose.
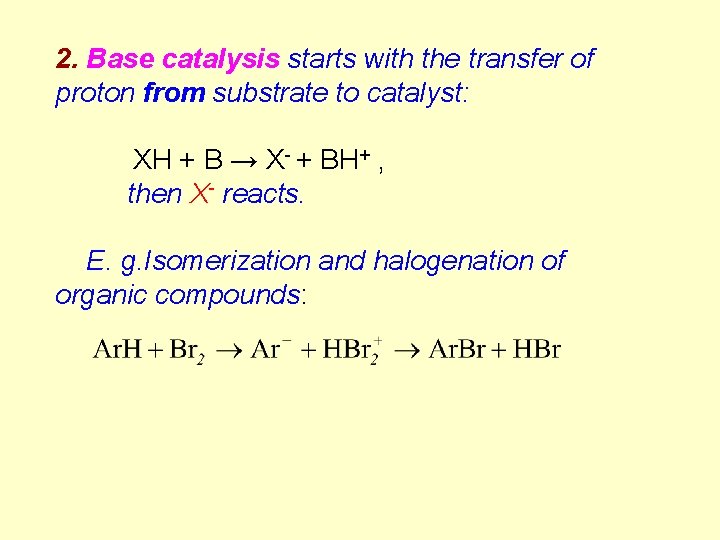
2. Base catalysis starts with the transfer of proton from substrate to catalyst: XH + B → X- + BH+ , then X- reacts. E. g. Isomerization and halogenation of organic compounds:
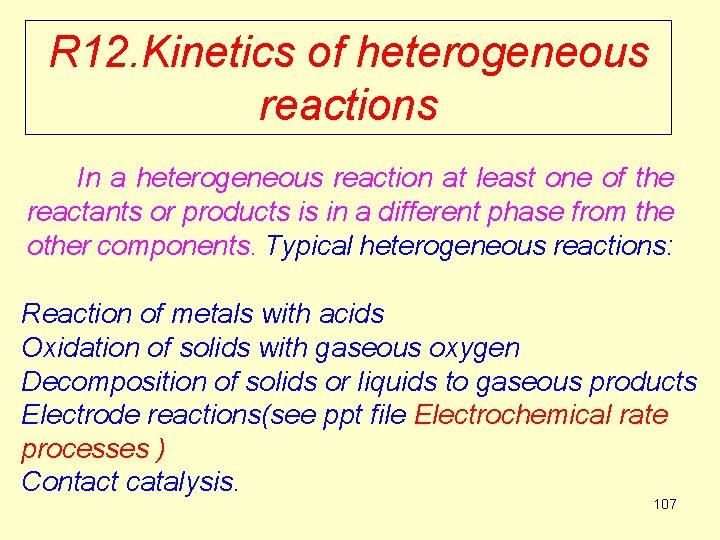
R 12. Kinetics of heterogeneous reactions In a heterogeneous reaction at least one of the reactants or products is in a different phase from the other components. Typical heterogeneous reactions: Reaction of metals with acids Oxidation of solids with gaseous oxygen Decomposition of solids or liquids to gaseous products Electrode reactions(see ppt file Electrochemical rate processes ) Contact catalysis. 107
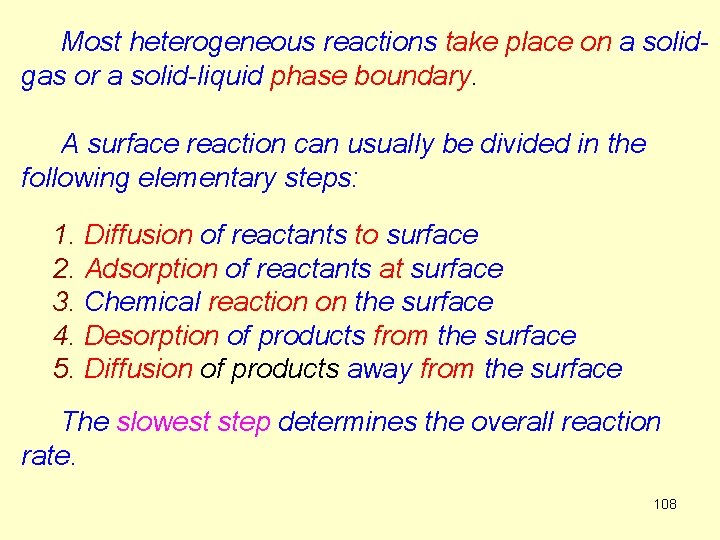
Most heterogeneous reactions take place on a solidgas or a solid-liquid phase boundary. A surface reaction can usually be divided in the following elementary steps: 1. Diffusion of reactants to surface 2. Adsorption of reactants at surface 3. Chemical reaction on the surface 4. Desorption of products from the surface 5. Diffusion of products away from the surface The slowest step determines the overall reaction rate. 108
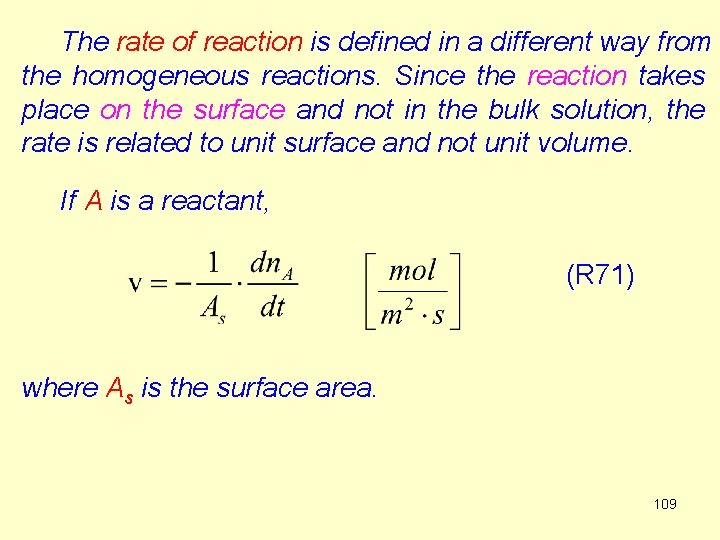
The rate of reaction is defined in a different way from the homogeneous reactions. Since the reaction takes place on the surface and not in the bulk solution, the rate is related to unit surface and not unit volume. If A is a reactant, (R 71) where As is the surface area. 109
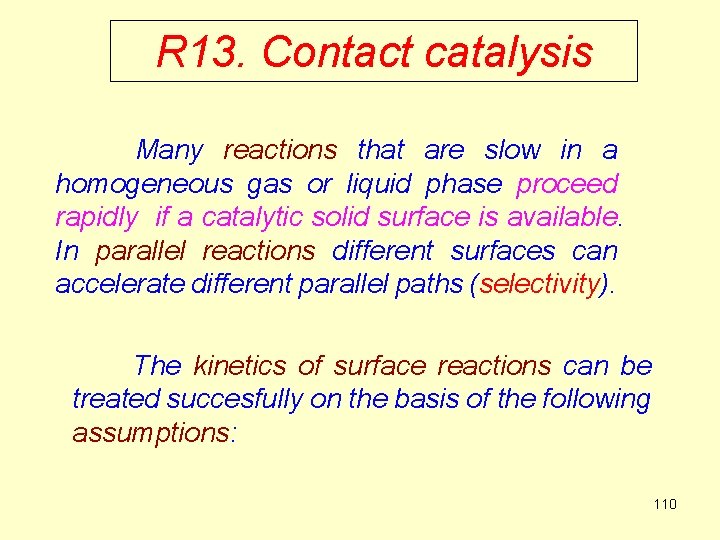
R 13. Contact catalysis Many reactions that are slow in a homogeneous gas or liquid phase proceed rapidly if a catalytic solid surface is available. In parallel reactions different surfaces can accelerate different parallel paths (selectivity). The kinetics of surface reactions can be treated succesfully on the basis of the following assumptions: 110
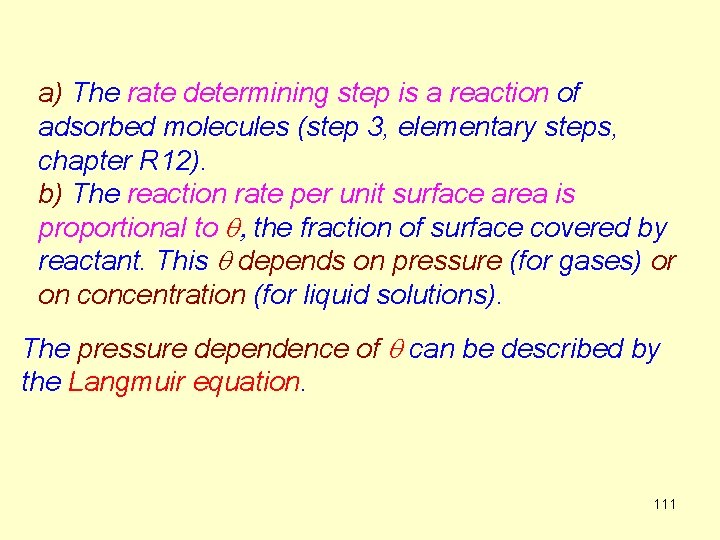
a) The rate determining step is a reaction of adsorbed molecules (step 3, elementary steps, chapter R 12). b) The reaction rate per unit surface area is proportional to q, the fraction of surface covered by reactant. This q depends on pressure (for gases) or on concentration (for liquid solutions). The pressure dependence of q can be described by the Langmuir equation. 111
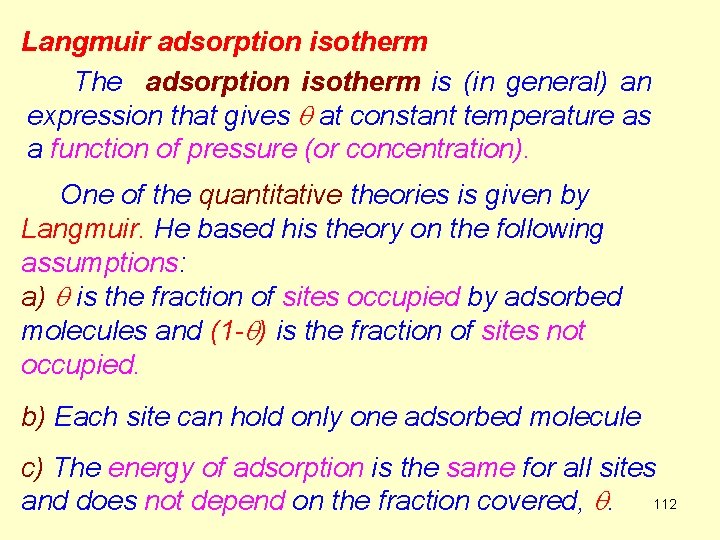
Langmuir adsorption isotherm The adsorption isotherm is (in general) an expression that gives q at constant temperature as a function of pressure (or concentration). One of the quantitative theories is given by Langmuir. He based his theory on the following assumptions: a) q is the fraction of sites occupied by adsorbed molecules and (1 -q) is the fraction of sites not occupied. b) Each site can hold only one adsorbed molecule c) The energy of adsorption is the same for all sites and does not depend on the fraction covered, q. 112
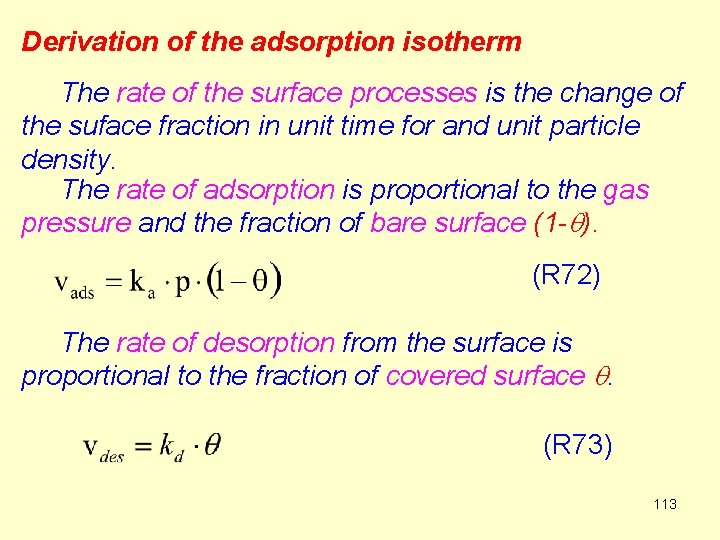
Derivation of the adsorption isotherm The rate of the surface processes is the change of the suface fraction in unit time for and unit particle density. The rate of adsorption is proportional to the gas pressure and the fraction of bare surface (1 -q). (R 72) The rate of desorption from the surface is proportional to the fraction of covered surface q. (R 73) 113
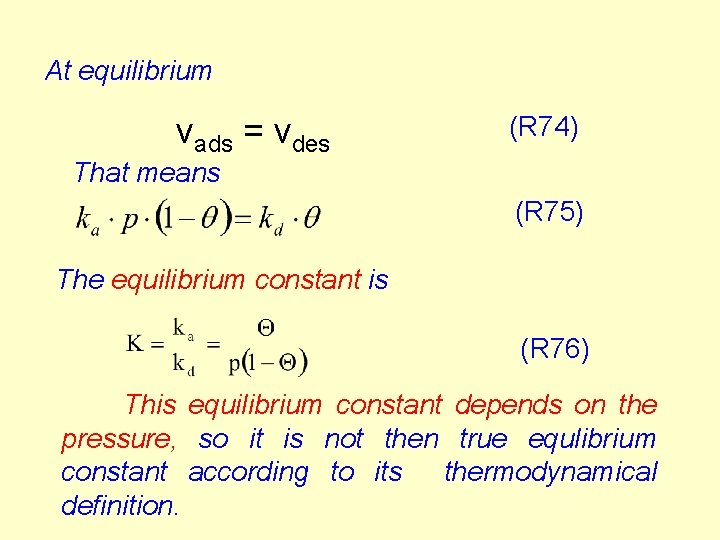
At equilibrium vads = vdes (R 74) That means (R 75) The equilibrium constant is (R 76) This equilibrium constant depends on the pressure, so it is not then true equlibrium constant according to its thermodynamical definition.
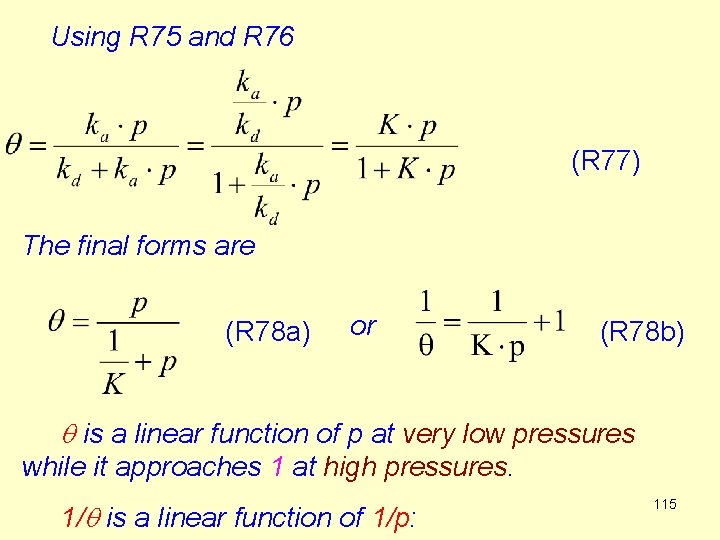
Using R 75 and R 76 (R 77) The final forms are (R 78 a) or (R 78 b) q is a linear function of p at very low pressures while it approaches 1 at high pressures. 1/q is a linear function of 1/p: 115
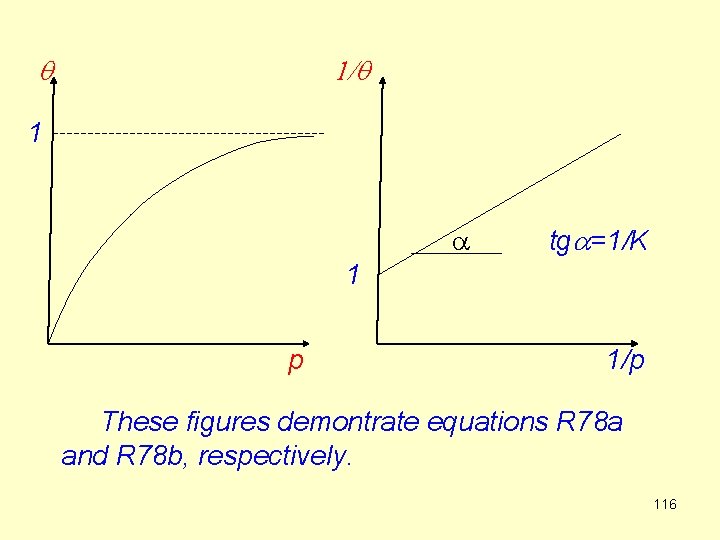
q 1/q 1 a tga=1/K 1 p 1/p These figures demontrate equations R 78 a and R 78 b, respectively. 116
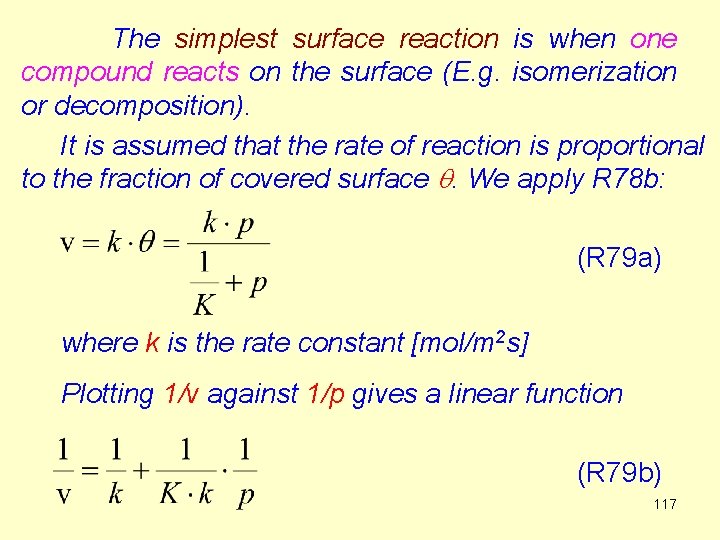
The simplest surface reaction is when one compound reacts on the surface (E. g. isomerization or decomposition). It is assumed that the rate of reaction is proportional to the fraction of covered surface q. We apply R 78 b: (R 79 a) where k is the rate constant [mol/m 2 s] Plotting 1/v against 1/p gives a linear function (R 79 b) 117
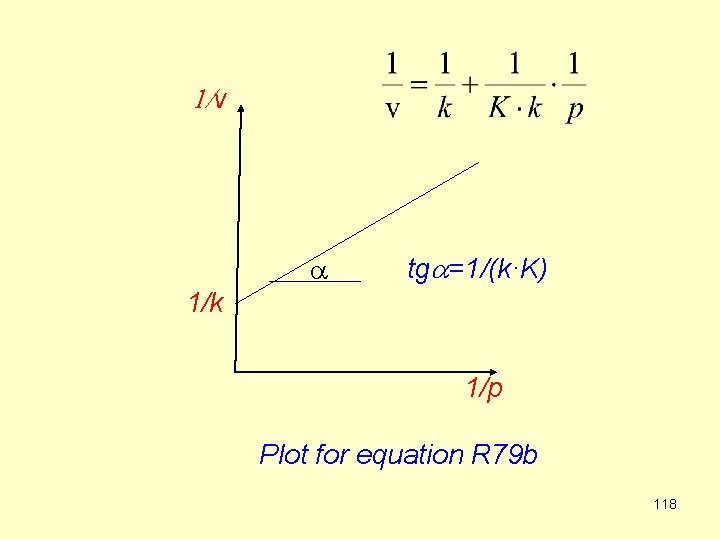
1/v a tga=1/(k·K) 1/k 1/p Plot for equation R 79 b 118
Reaction order
Kinetics reaction
Addition reaction and substitution reaction
Leukoerythroblastic reaction vs leukemoid reaction
Half-life formula
How does temperature affect rate of reaction
How to find the rate law of a reaction
How to write reaction rate
Reaction rate constant
Reactants leftover when a reaction stops are
How to determine the rate law of a reaction
Half life for second order reaction
How to calculate instantaneous rate of reaction
Rates of reaction quiz
Overall rate law of a reaction
Factors affecting the rate of chemical reaction temperature
Overall rate law of a reaction
How to determine the rate law of a reaction
Laws jespersen
Surface area affecting rate of reaction
What is catalyst and how it affects reaction rate
How to calculate rate of reaction
Determine activation energy from graph
Finding initial rate of reaction
Reaction rate and stoichiometry
Equilibrium reaction rate
Cell kinetics and fermenter design
Data kinetics ltd
Kinetics of particles newton's second law
Octet biosensors
Kinetics half life
First order drug elimination
Growth kinetics
Enzyme kinetics
Kinetics of a particle: force and acceleration
Collision theory of kinetics
Kcat
Enzyme catalyze
Chemical kinetics experiment
Chemical kinetics definition
Plane kinetics of rigid bodies
Steady state approximation examples
Vampnets for deep learning of molecular kinetics
Abbas et al
Ap chem kinetics
Definition of chemical kinetics in chemistry
Chemistry grade 11 unit 4 chemical kinetics
Planar kinetics of a rigid body work and energy
Km in enzyme kinetics
Kinetics of a particle: impulse and momentum
Planar kinetics of a rigid body force and acceleration
Difference between zero and first order kinetics
Kinetics is the branch of:
Kinematics of rigid bodies problems and solutions
Kinetics of crystal violet fading
Kinetics and equilibrium
Kinetics flotation chemicals
Applications of chemical kinetics
Dynafit kinetics
Kinetics of a rigid body
Zero order elimination drugs
What is real interest rate and nominal interest rate
1 year forward rate formula
Growth analysis
Spot rate and forward rate
Contoh transaksi forward
What is the difference between rate and unit rate
Cap rate interest rate relationship
Addison currency exchange
Dạng đột biến một nhiễm là
Vẽ hình chiếu đứng bằng cạnh của vật thể
Phản ứng thế ankan
Voi kéo gỗ như thế nào
Các môn thể thao bắt đầu bằng từ đua
Sự nuôi và dạy con của hươu
Thiếu nhi thế giới liên hoan
điện thế nghỉ
Một số thể thơ truyền thống
Biện pháp chống mỏi cơ
Trời xanh đây là của chúng ta thể thơ
Lp html
Số nguyên là gì
Fecboak
Các châu lục và đại dương trên thế giới
Chụp phim tư thế worms-breton
Thế nào là hệ số cao nhất
Sơ đồ cơ thể người
Tư thế ngồi viết
Cái miệng bé xinh thế chỉ nói điều hay thôi
đặc điểm cơ thể của người tối cổ
Mật thư tọa độ 5x5
Bổ thể
Tư thế ngồi viết
ưu thế lai là gì
Thẻ vin
Thơ thất ngôn tứ tuyệt đường luật
Các châu lục và đại dương trên thế giới
Từ ngữ thể hiện lòng nhân hậu
Diễn thế sinh thái là
Vẽ hình chiếu vuông góc của vật thể sau
Thứ tự các dấu thăng giáng ở hóa biểu
Làm thế nào để 102-1=99
Tỉ lệ cơ thể trẻ em
Chúa yêu trần thế alleluia
Lời thề hippocrates
Sự nuôi và dạy con của hươu
đại từ thay thế
Quá trình desamine hóa có thể tạo ra
Cong thức tính động năng
Thế nào là mạng điện lắp đặt kiểu nổi
Hình ảnh bộ gõ cơ thể búng tay
2 amino acids joined together
Arrhenius reaction example
Supply chain synchronization
How common is leukemia in adults
Combustion reaction
Stereoselective and stereospecific reactions
Diene dienophile reaction