Complementary MOS inverter CMOS inverter Complementary MOS inverter
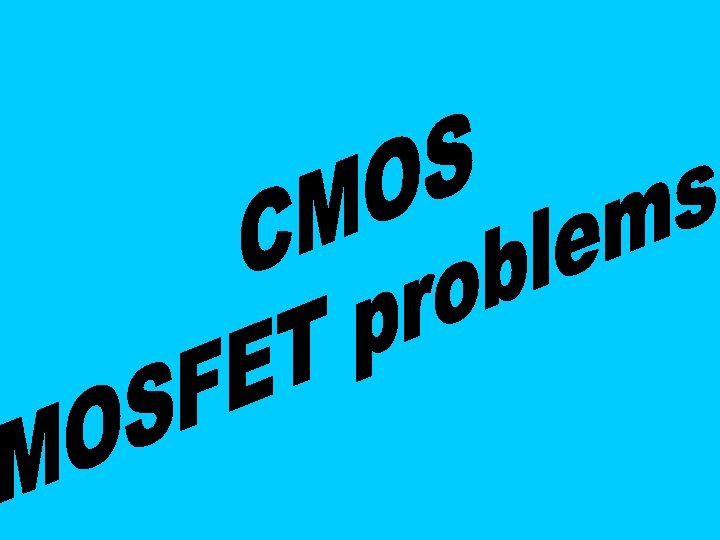
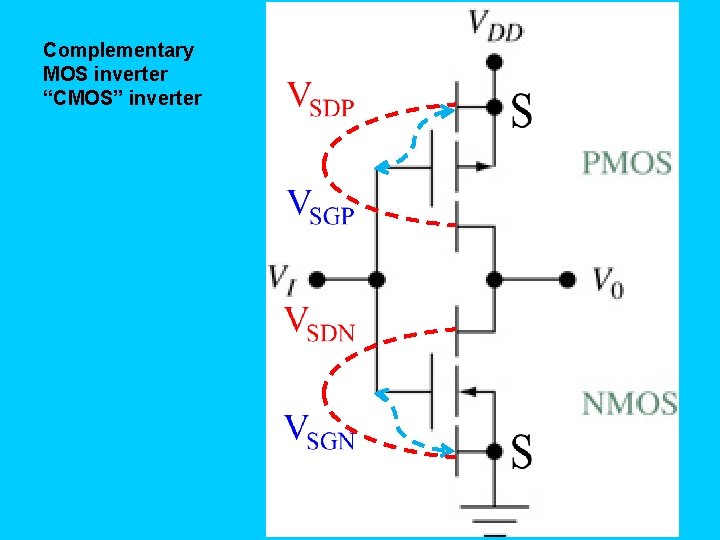
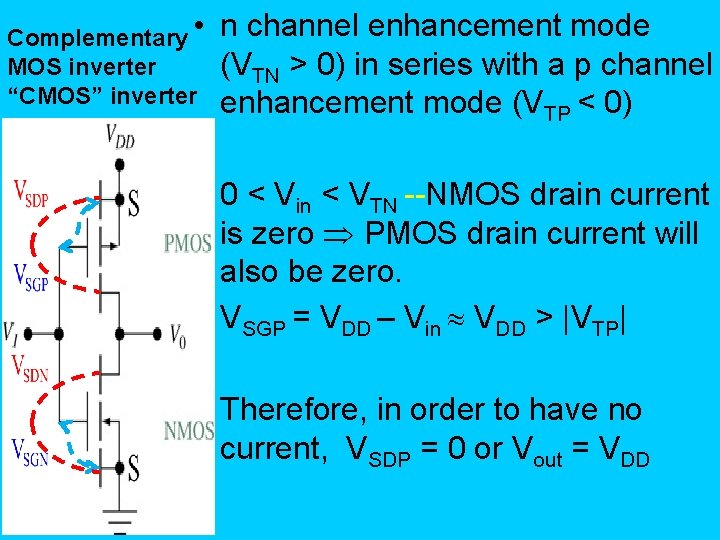
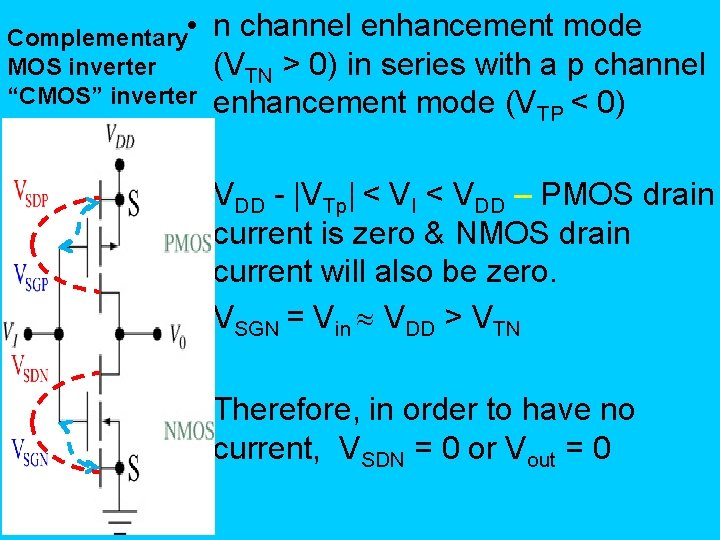
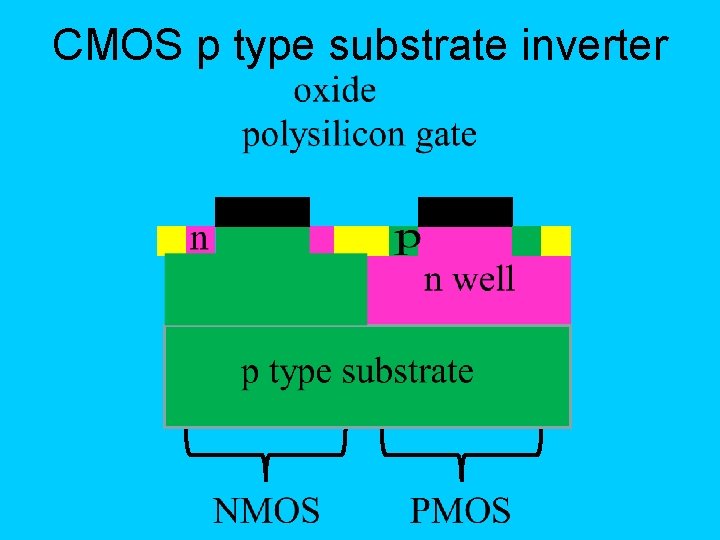
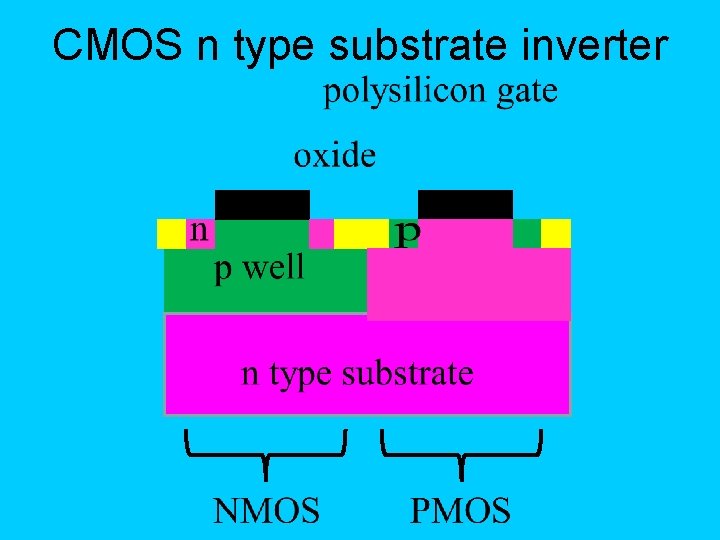
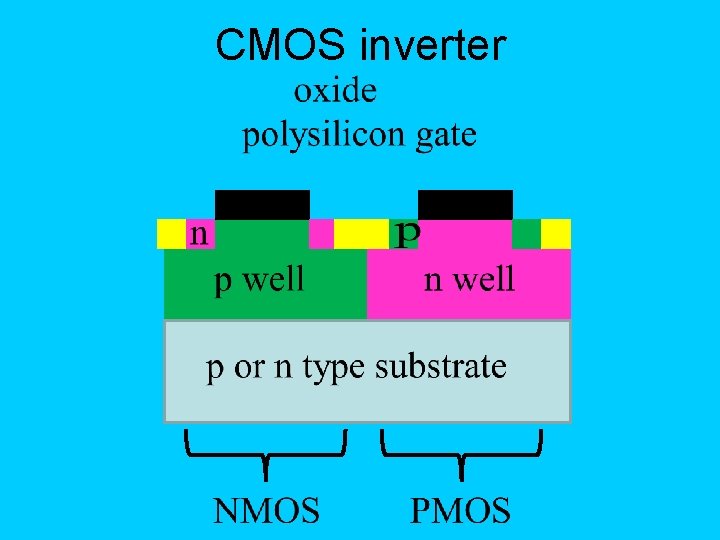
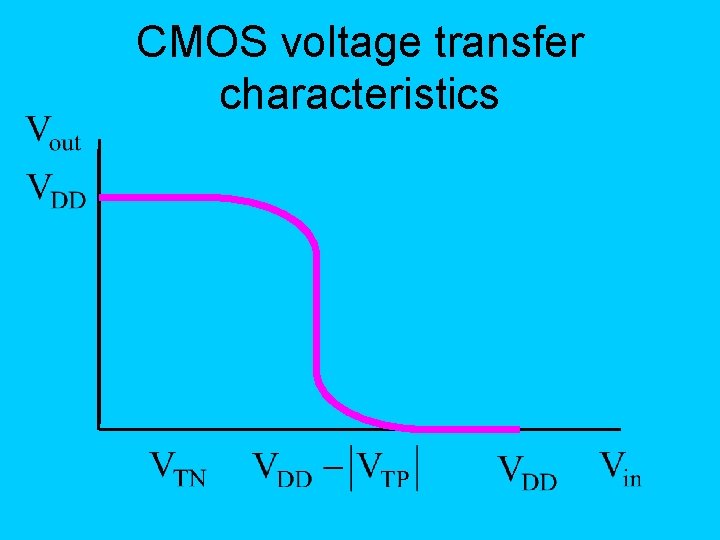
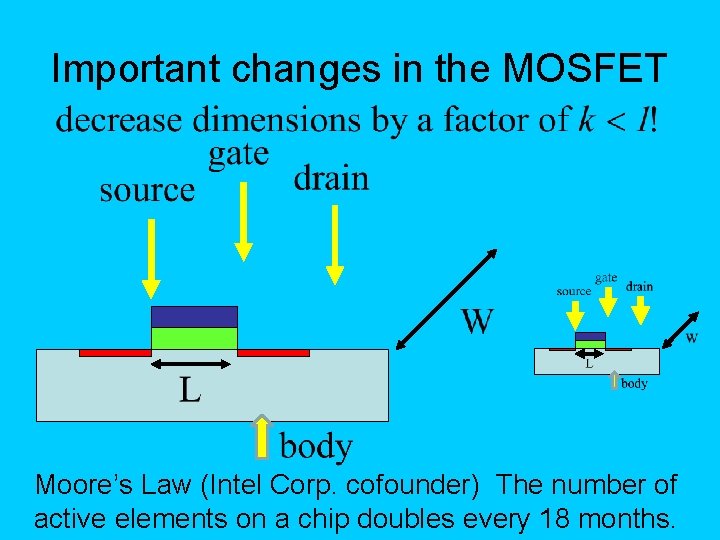
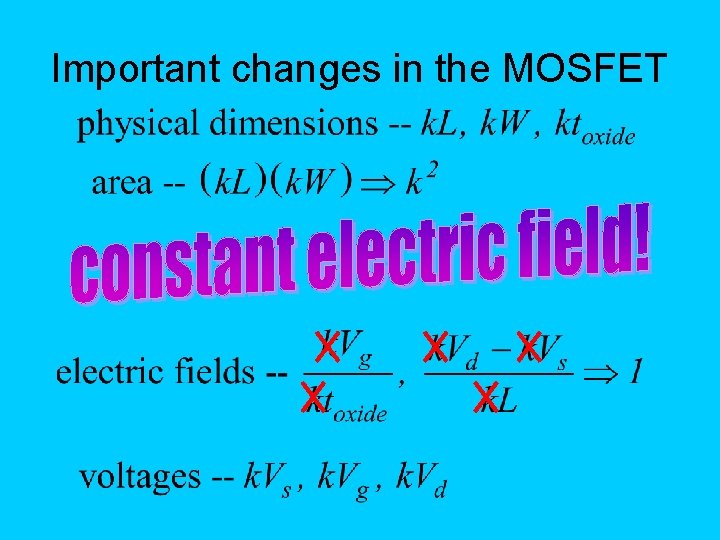
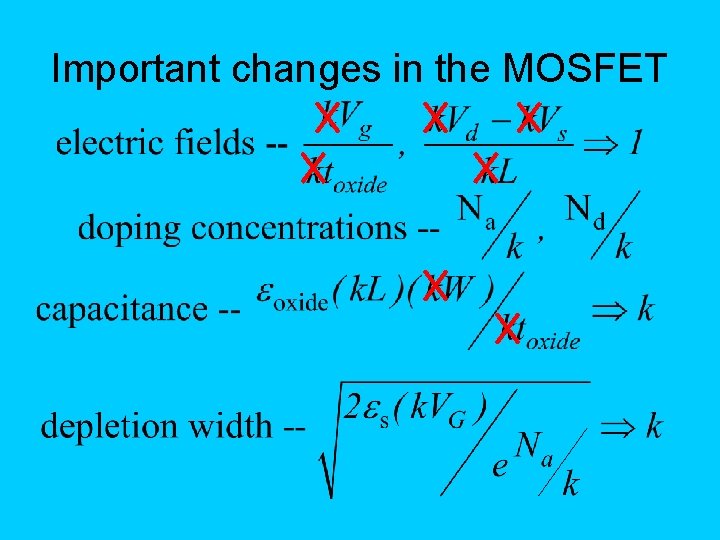
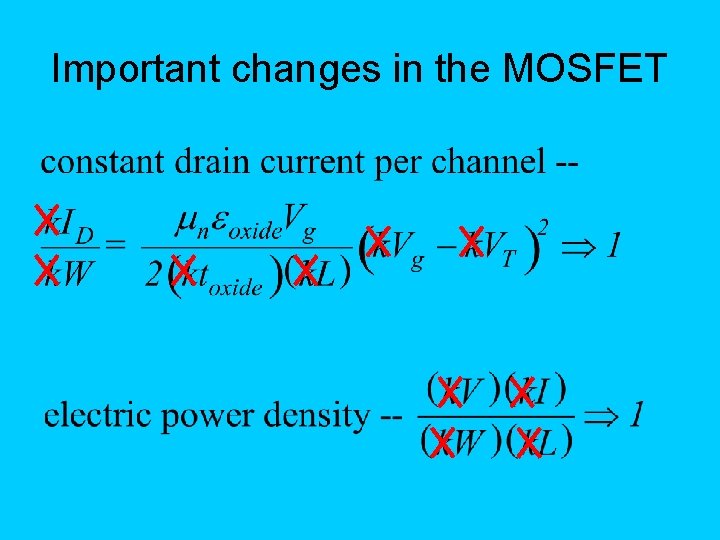
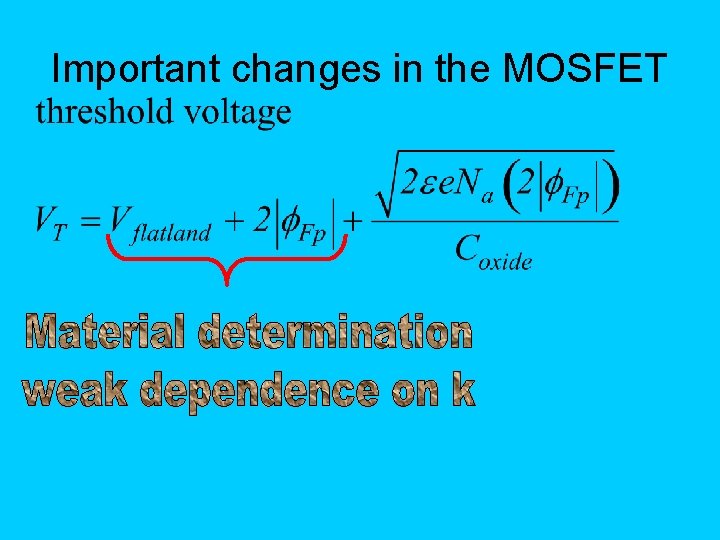
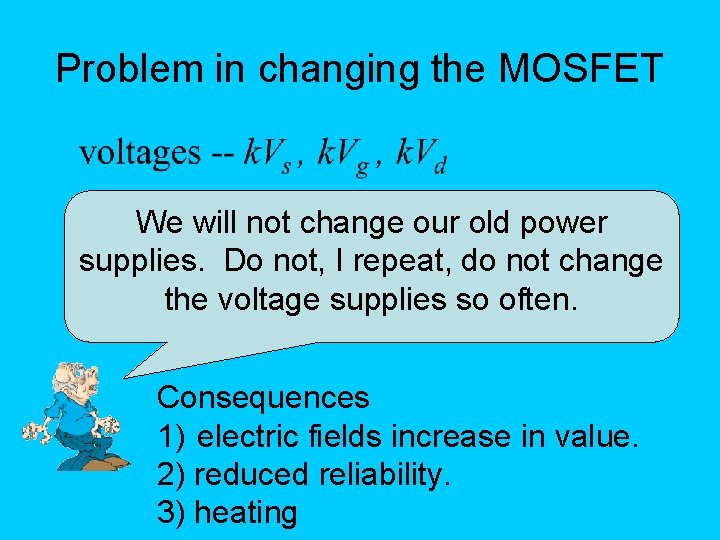
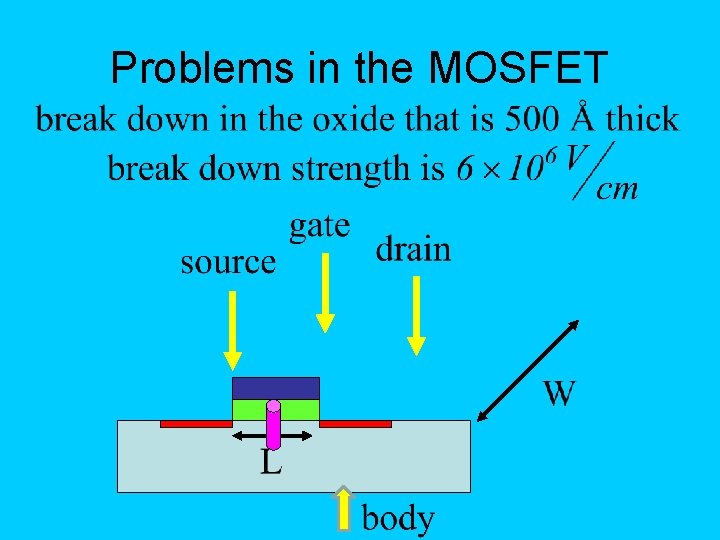
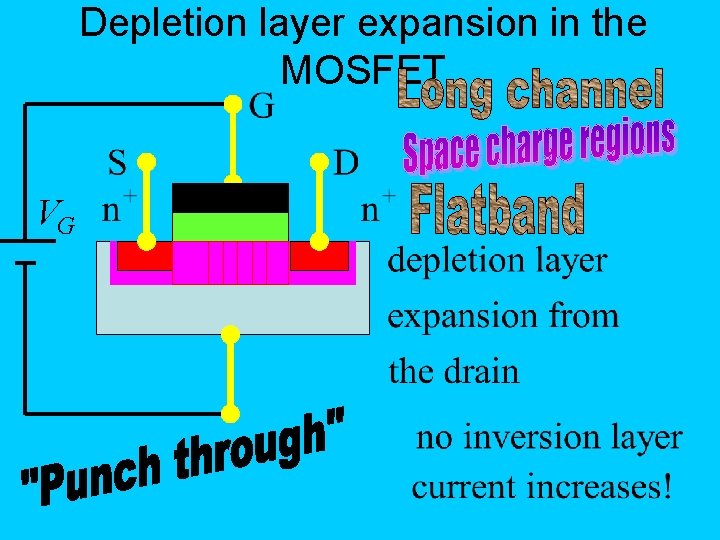
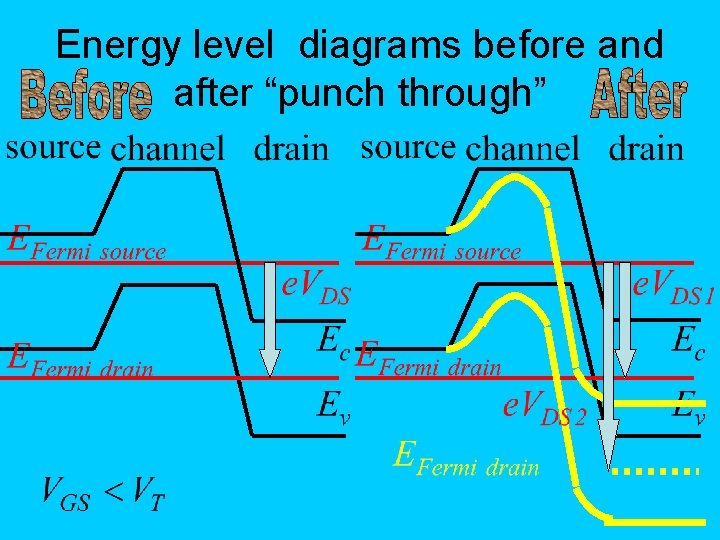
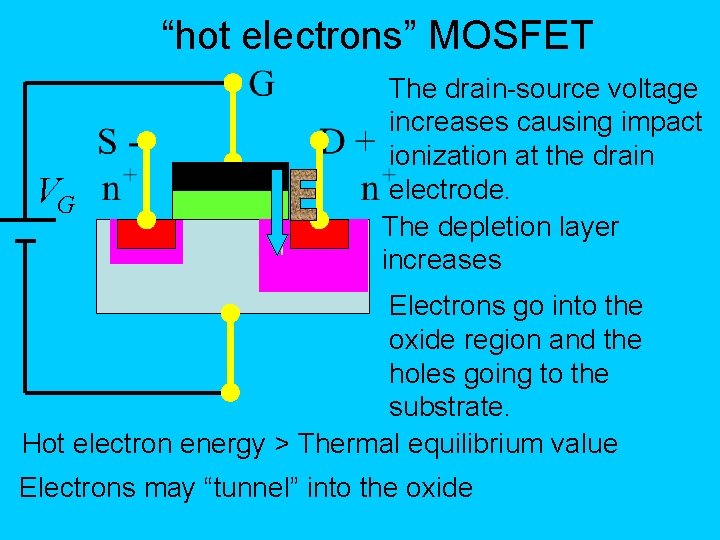
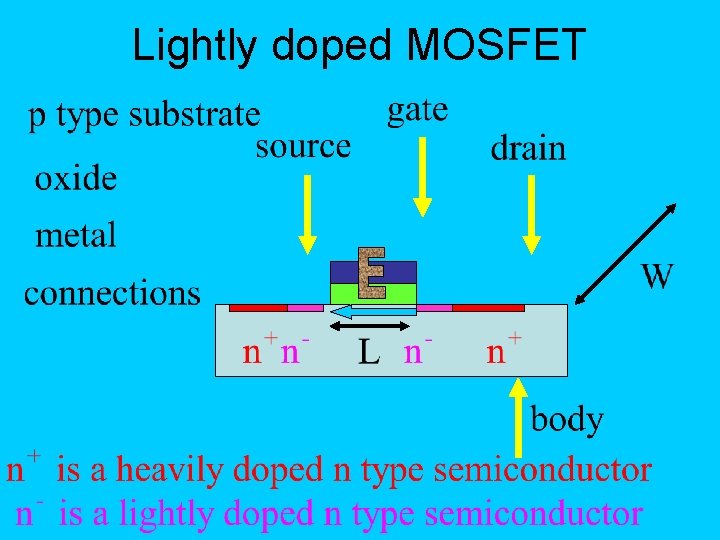
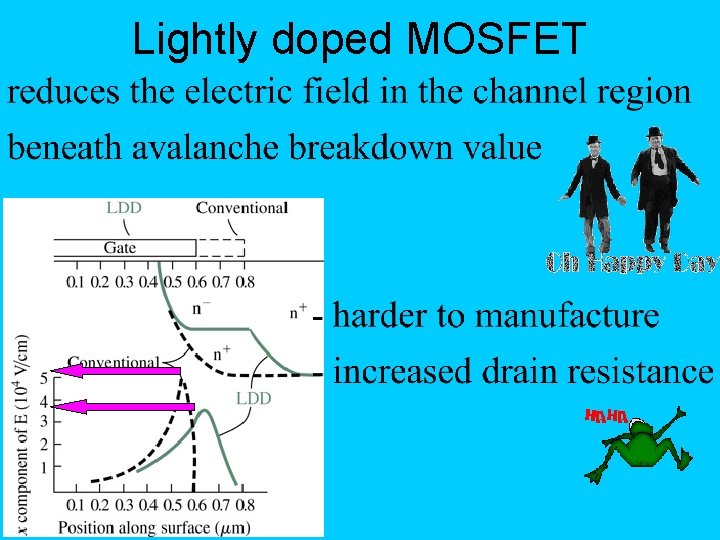
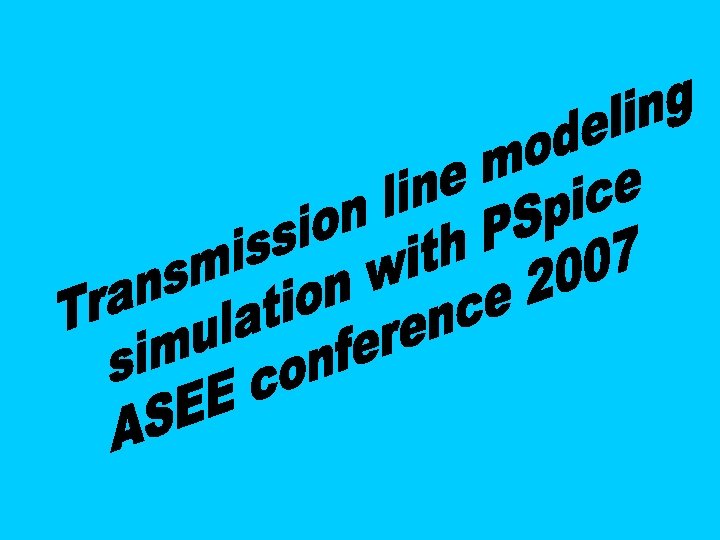
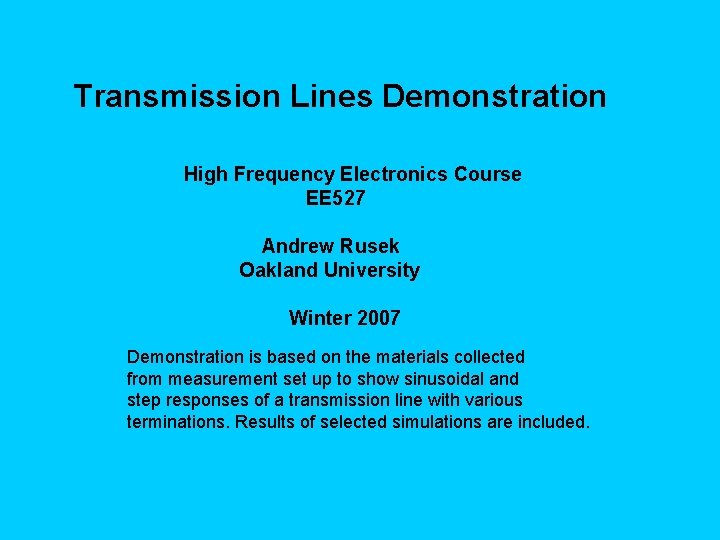
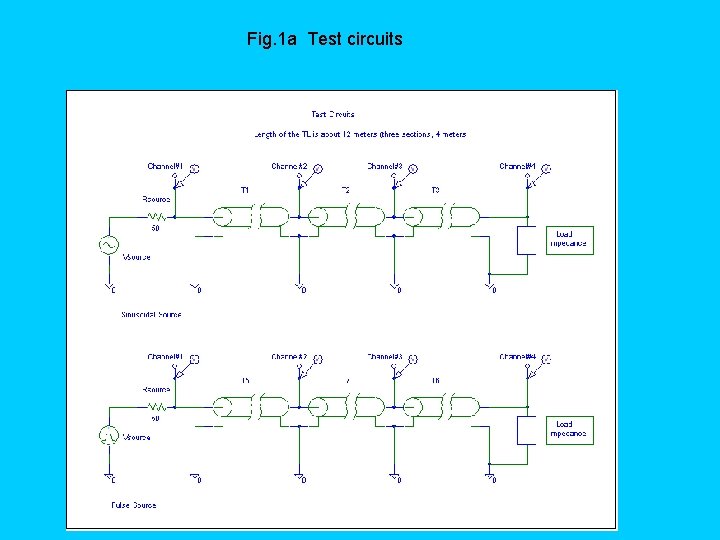
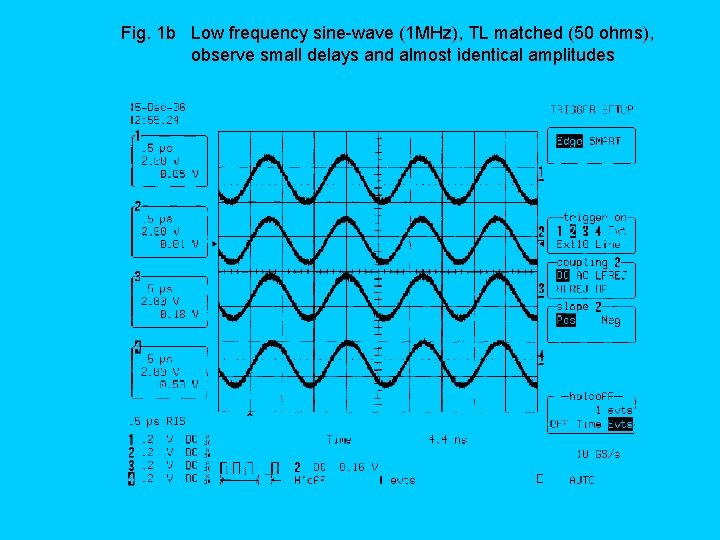
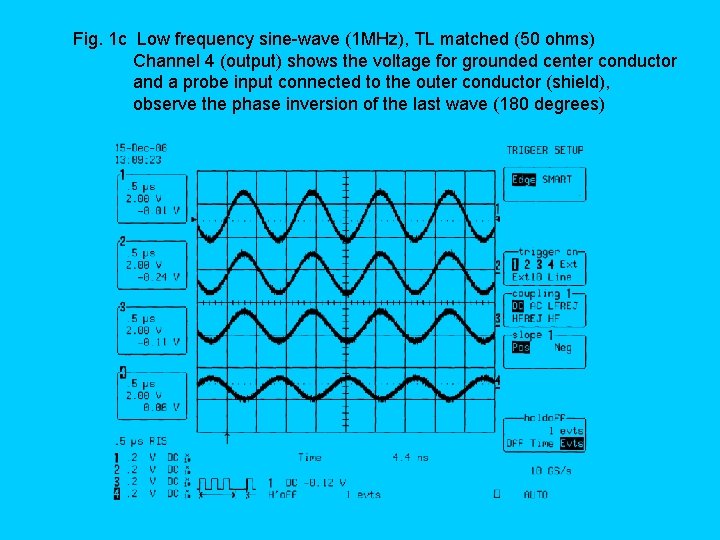
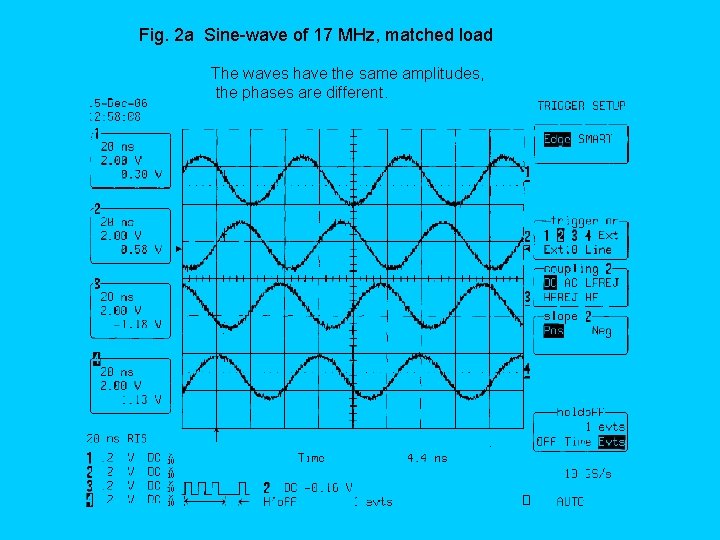
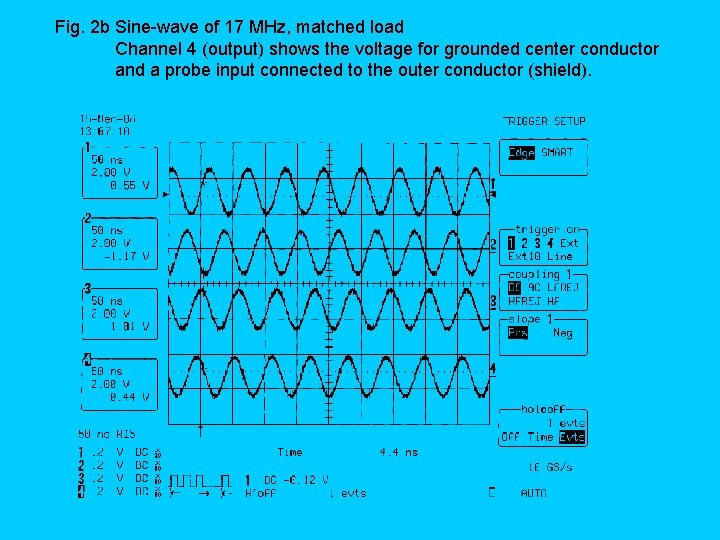
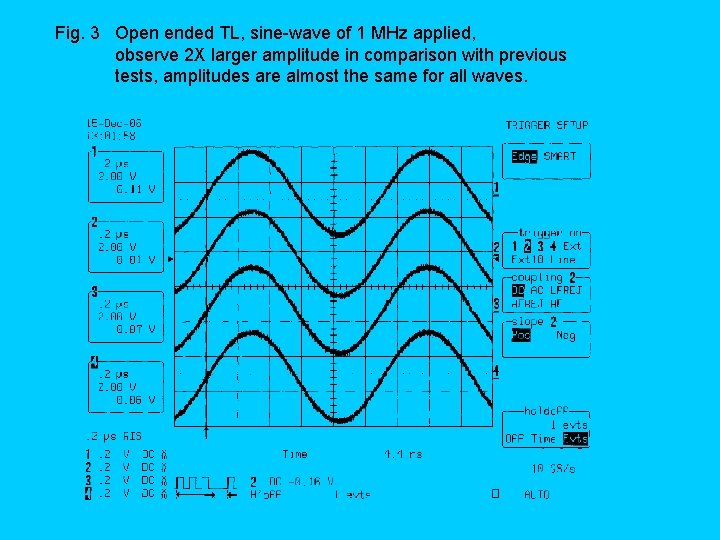
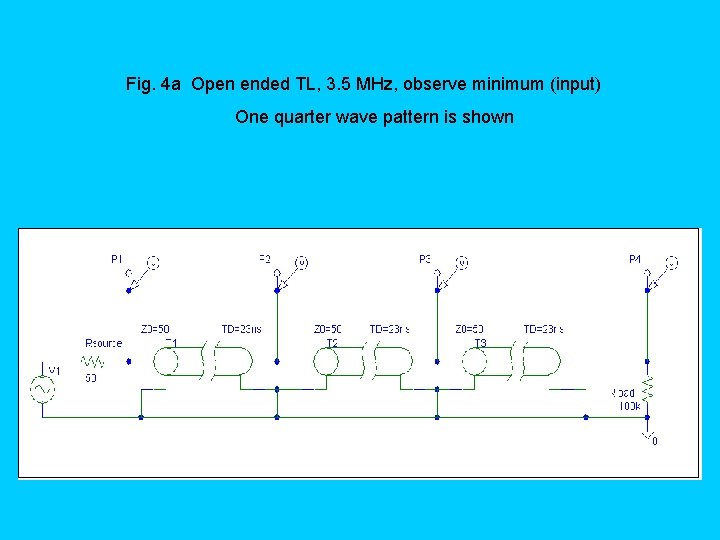
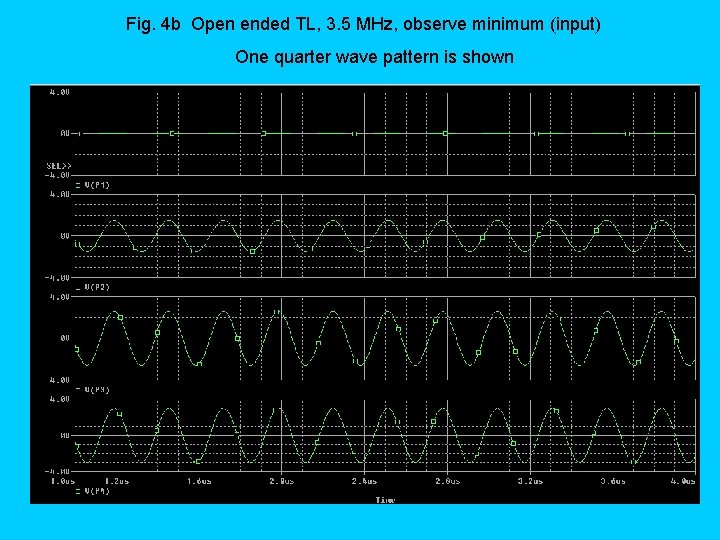
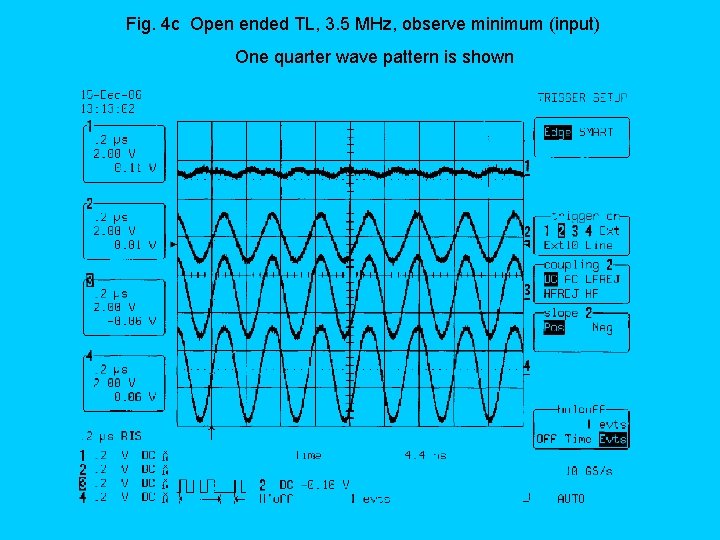
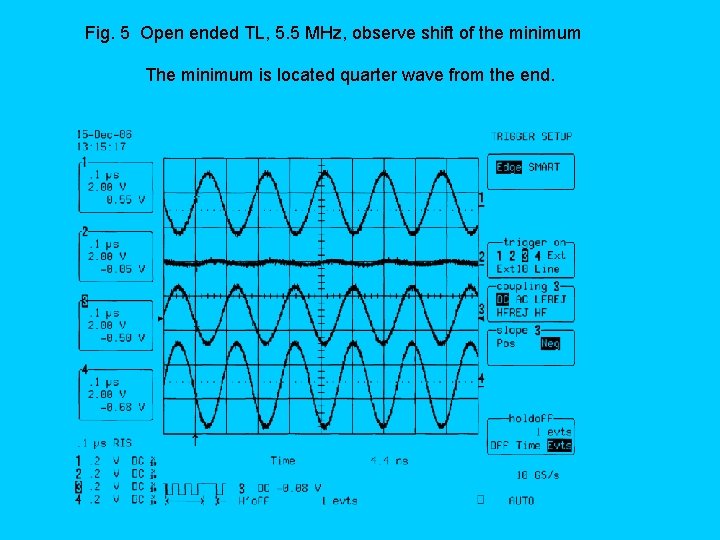
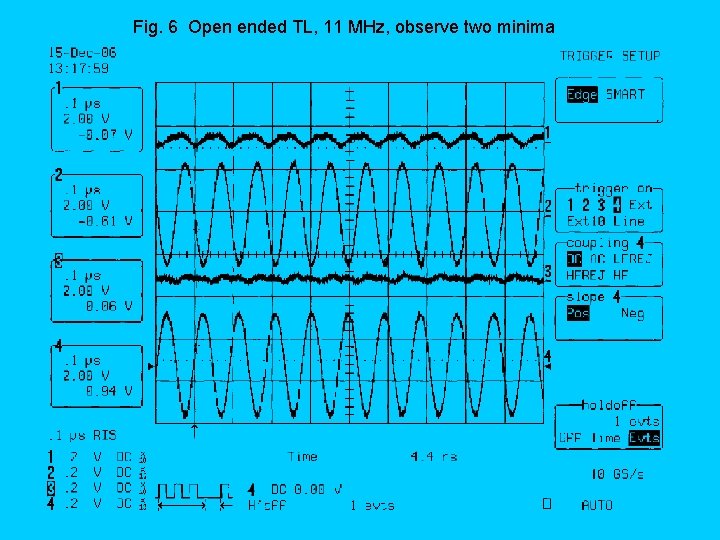
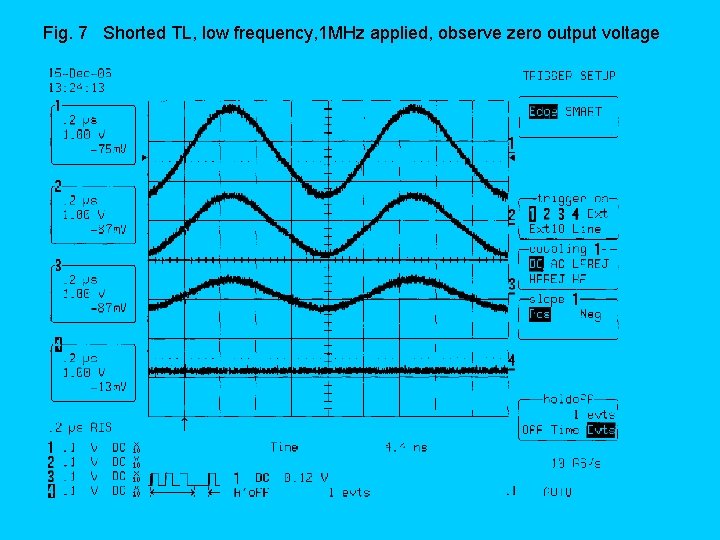
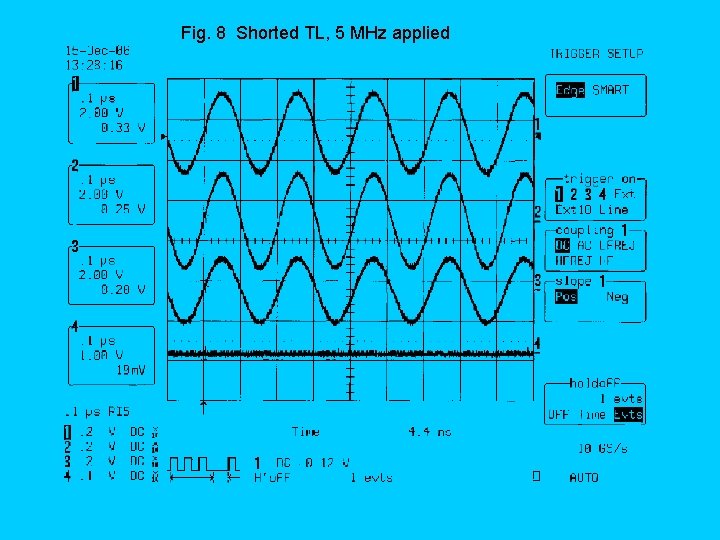
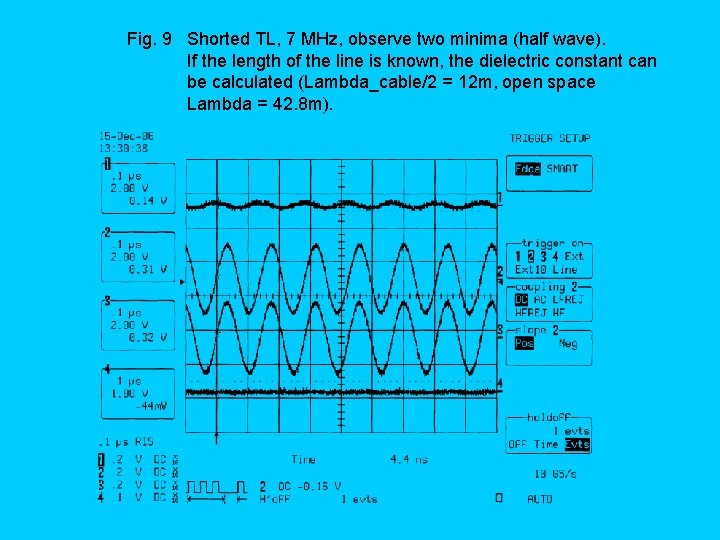
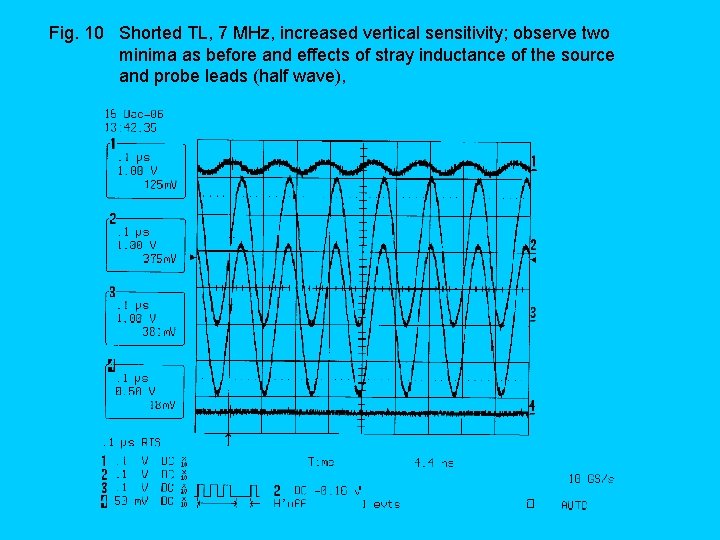
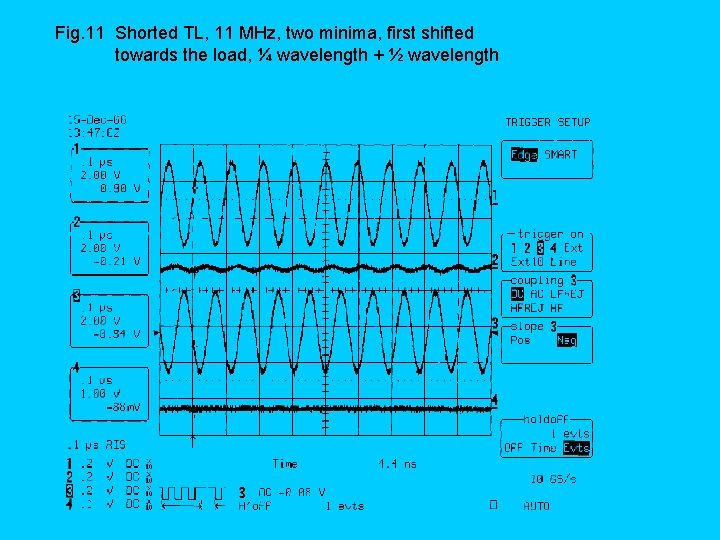
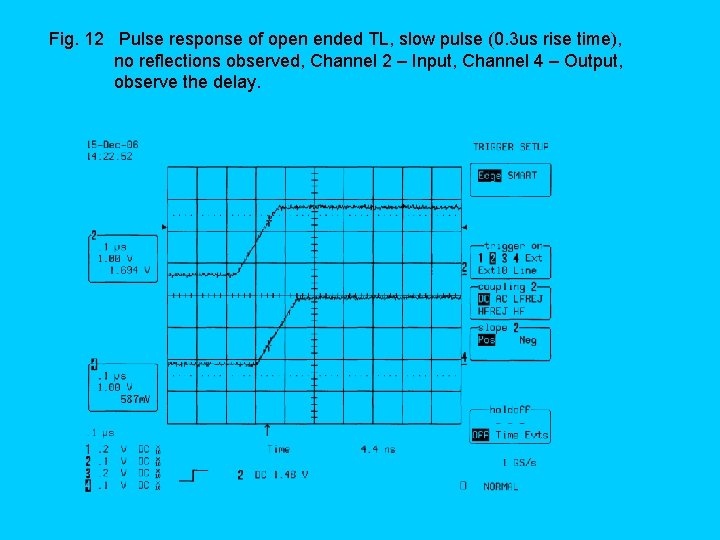
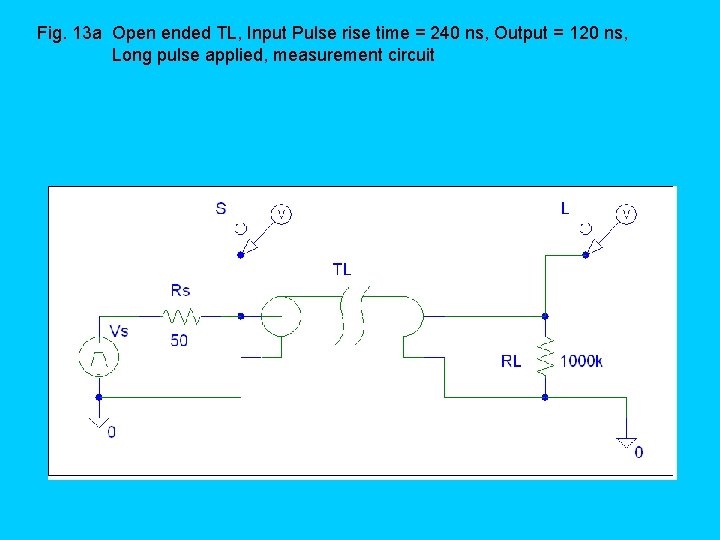
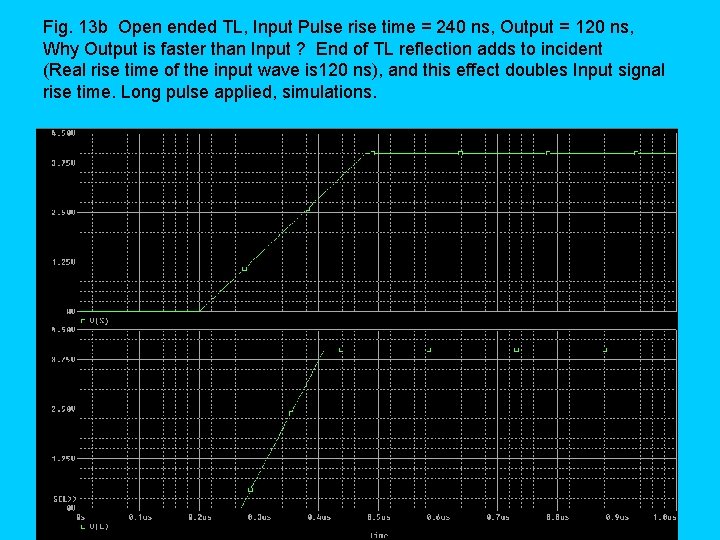
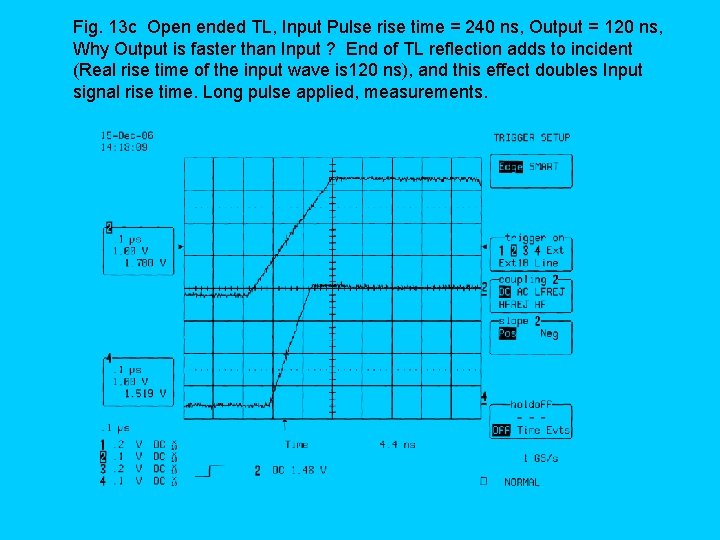
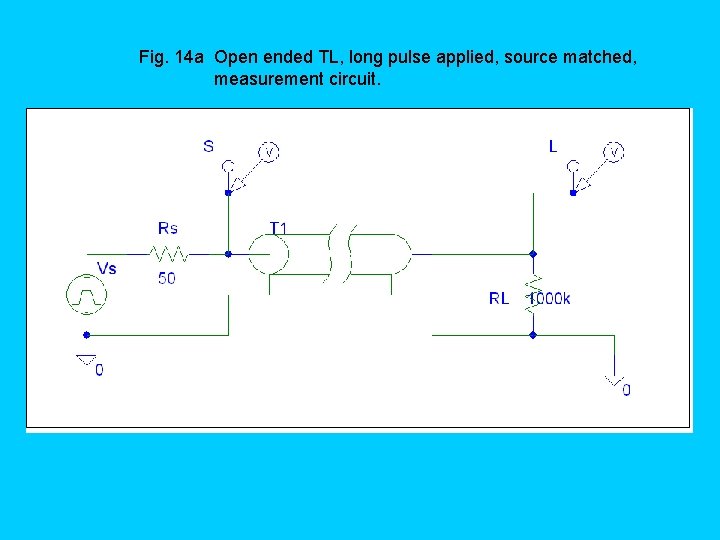
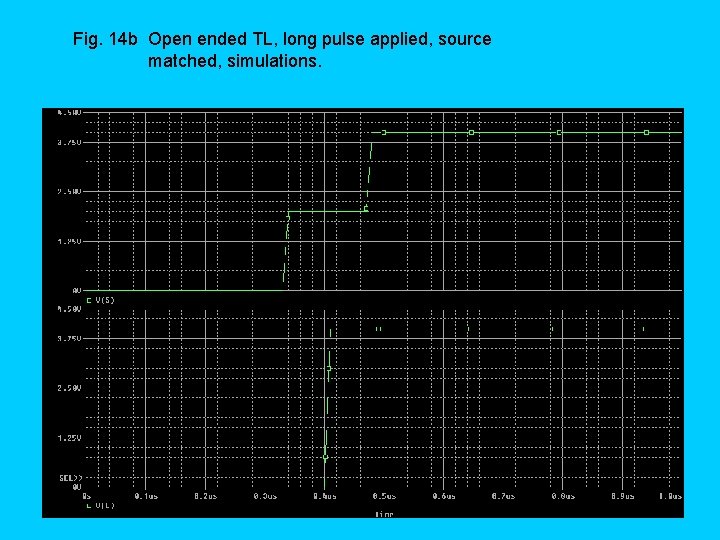
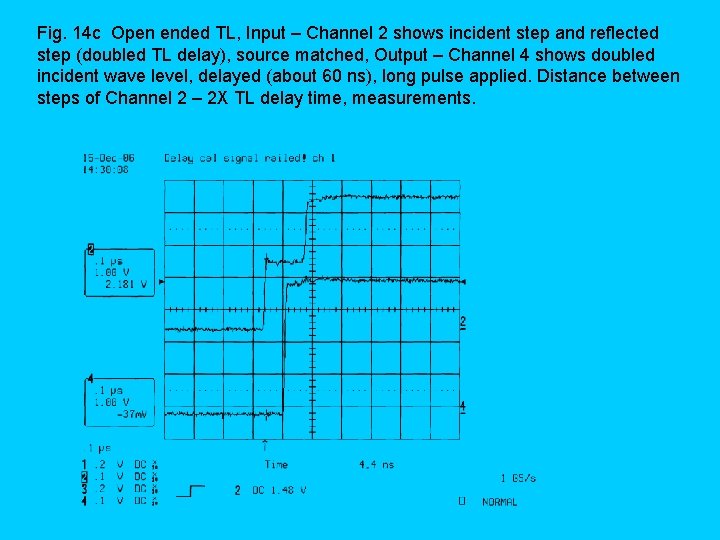
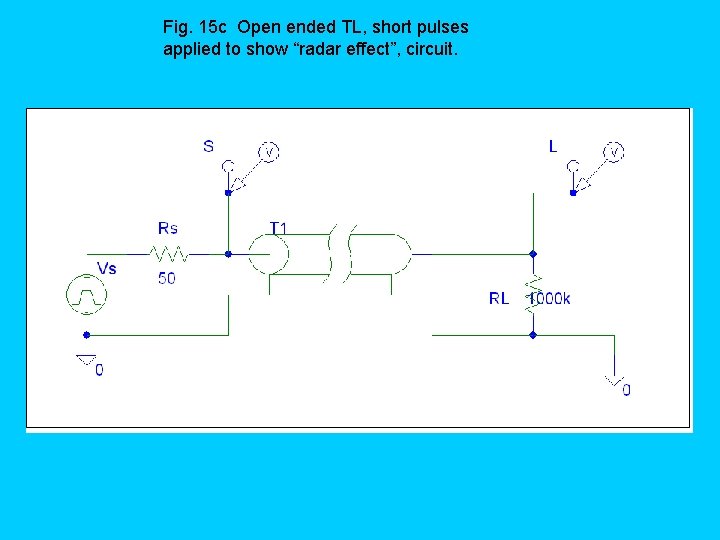
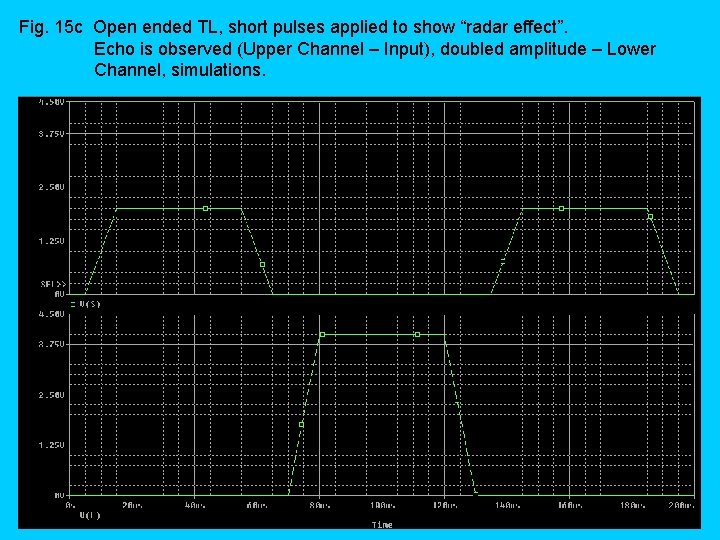
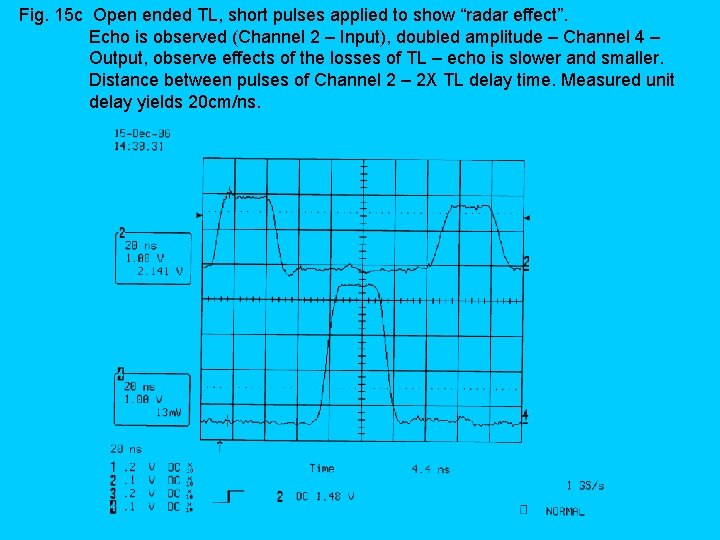
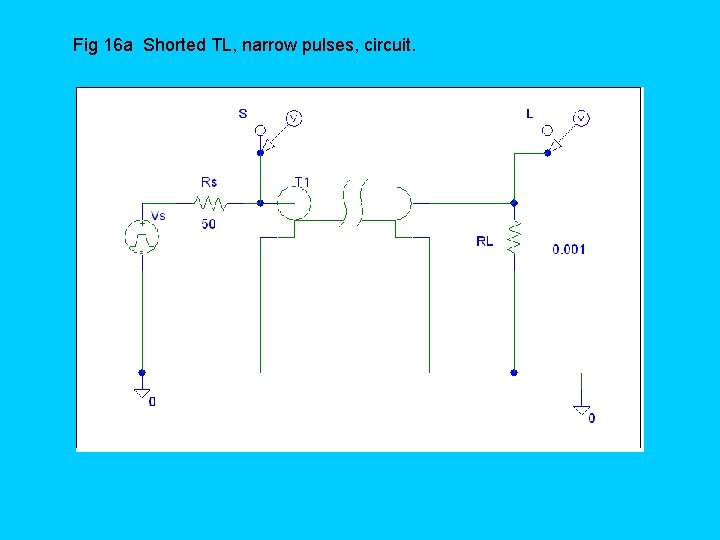
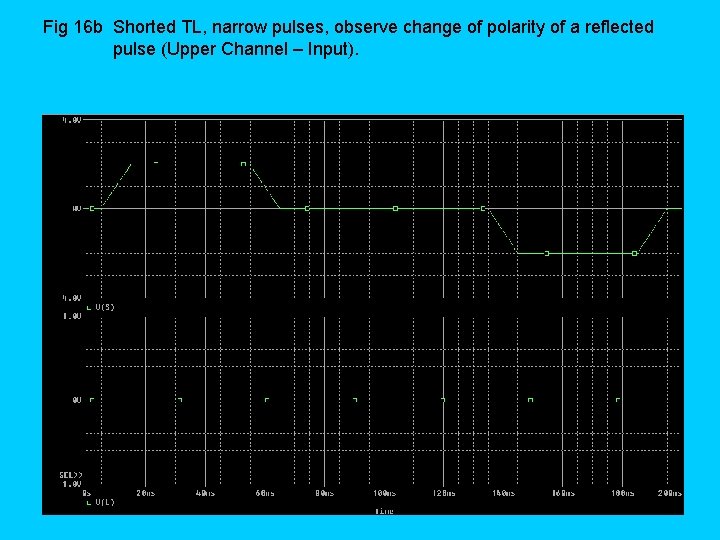
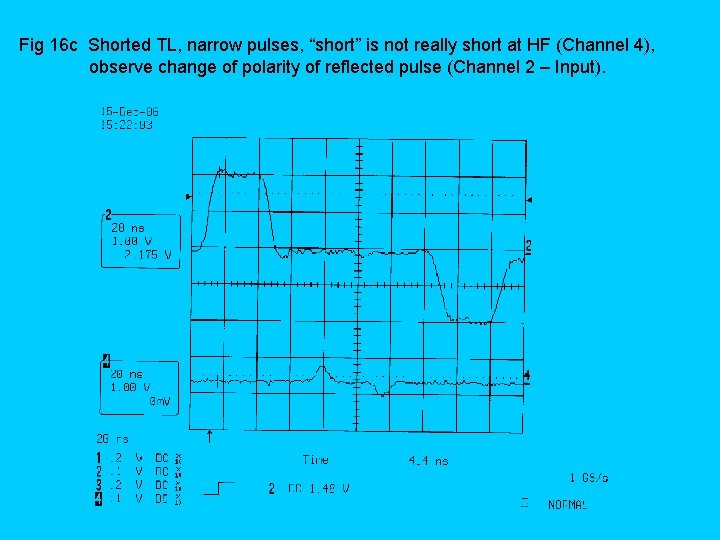
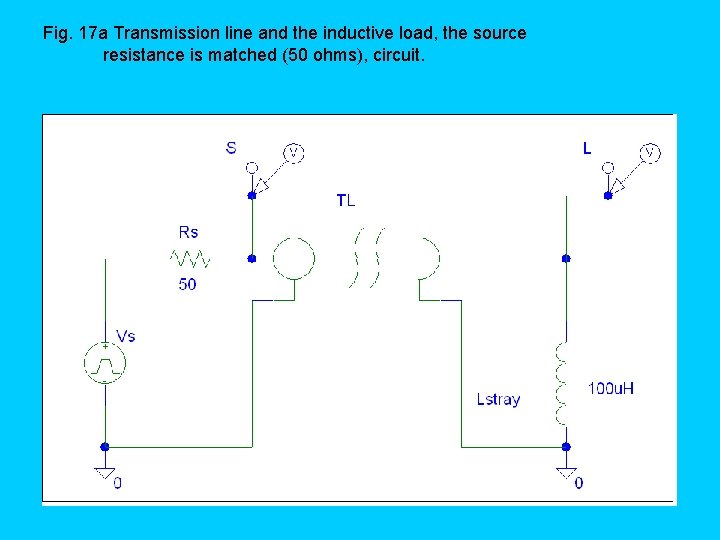
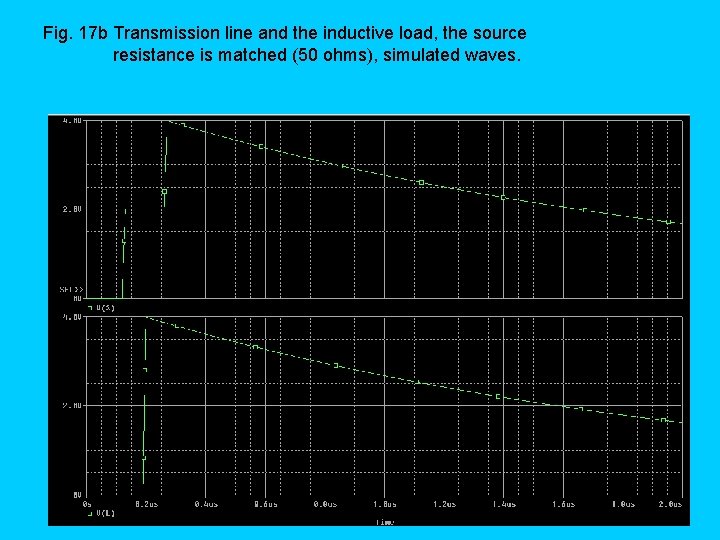
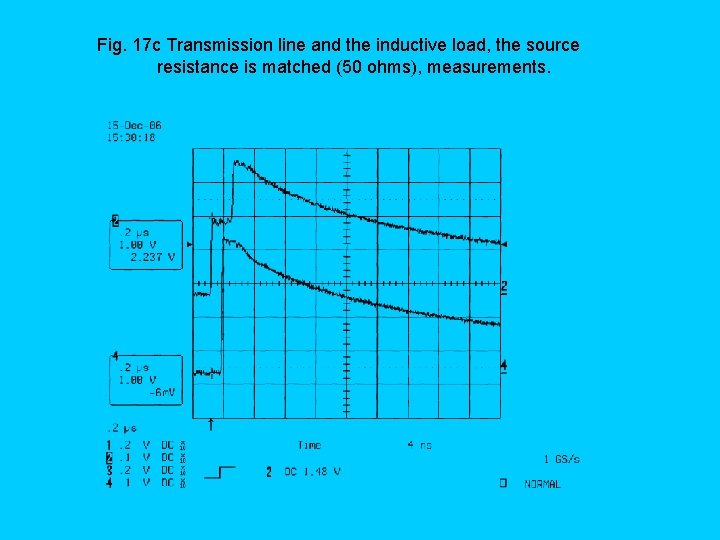
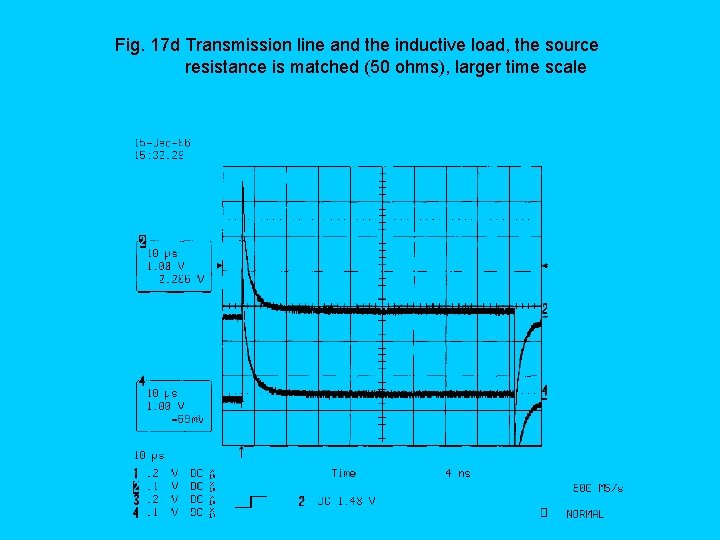
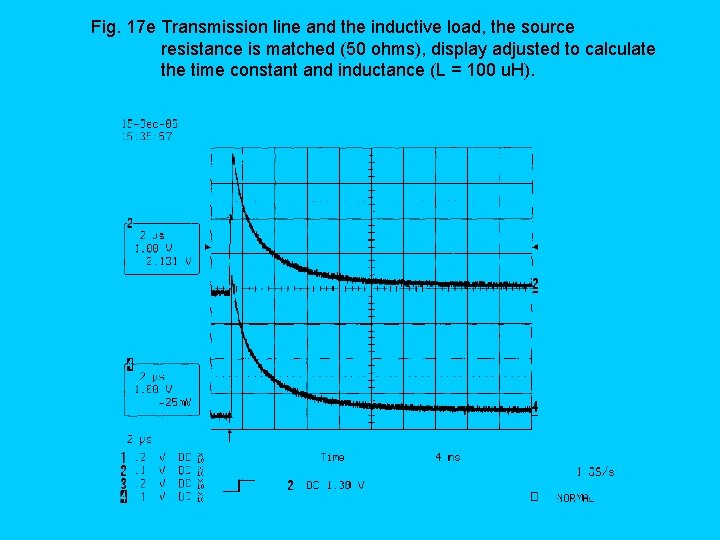
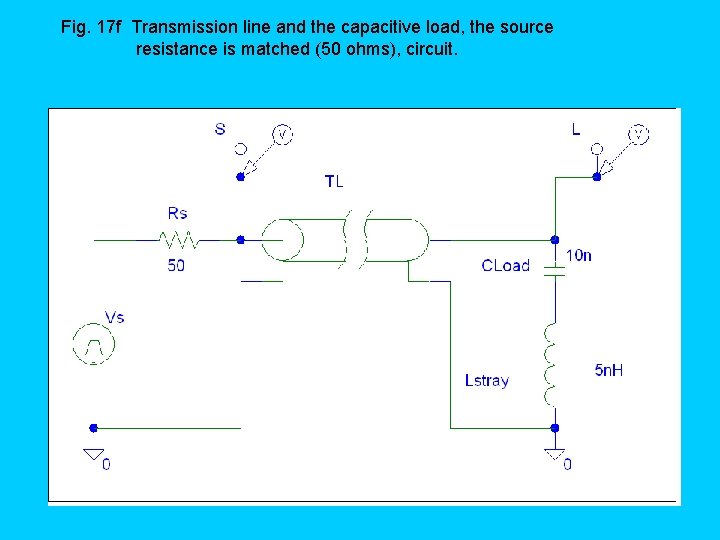
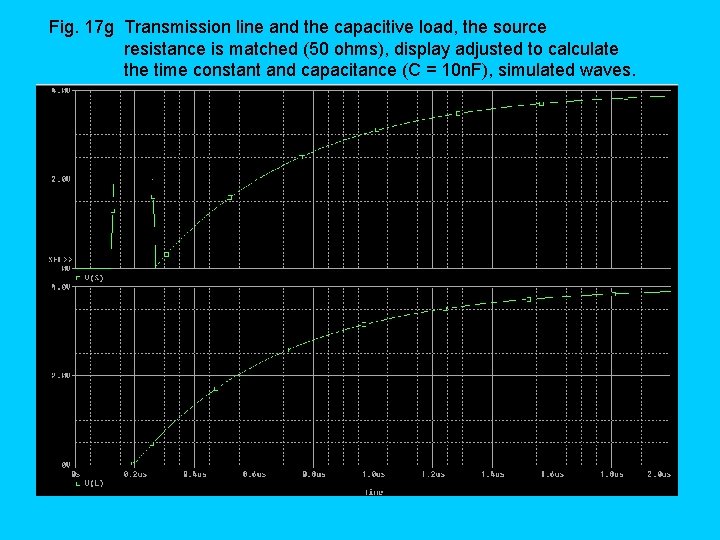
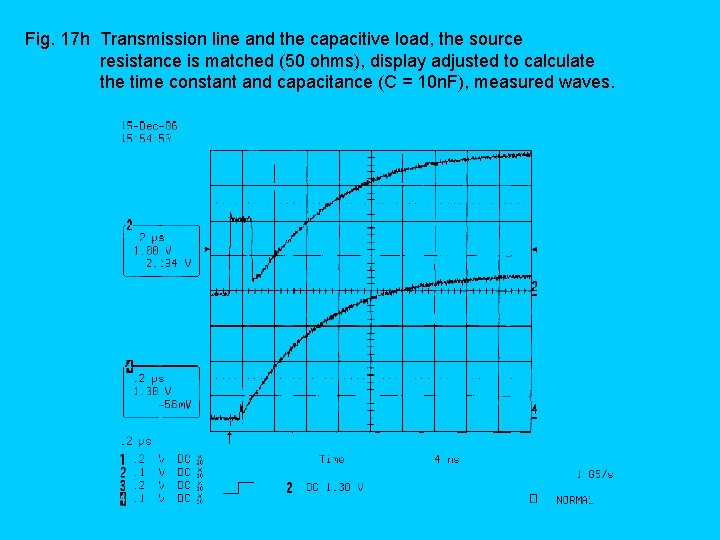
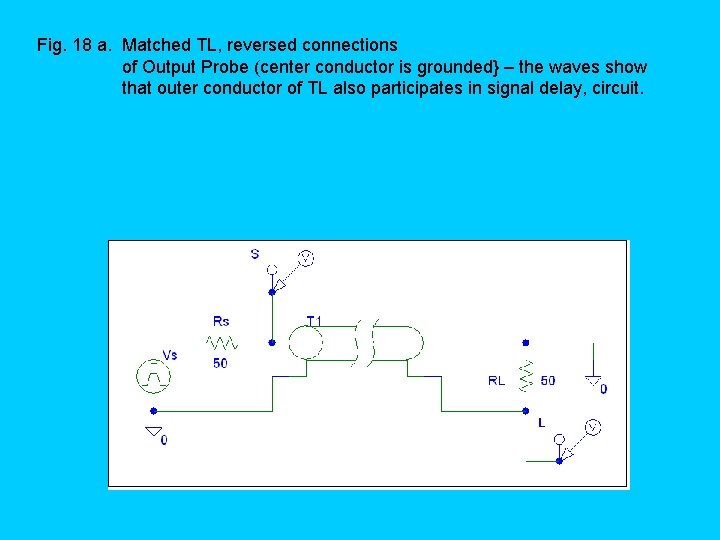
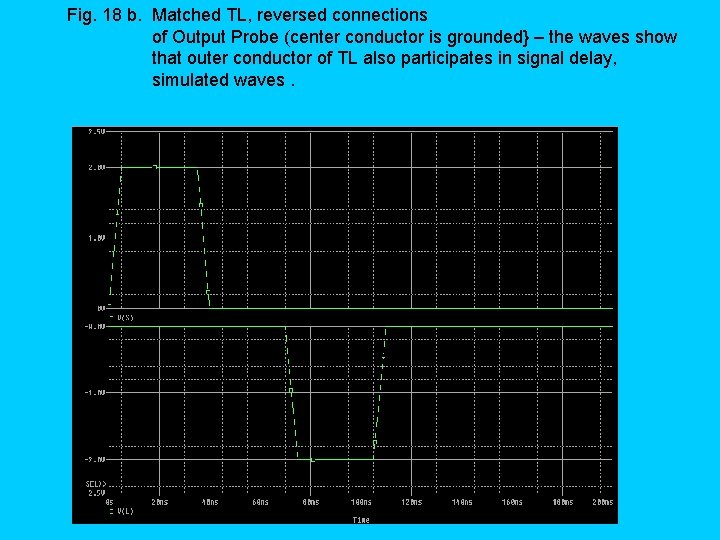
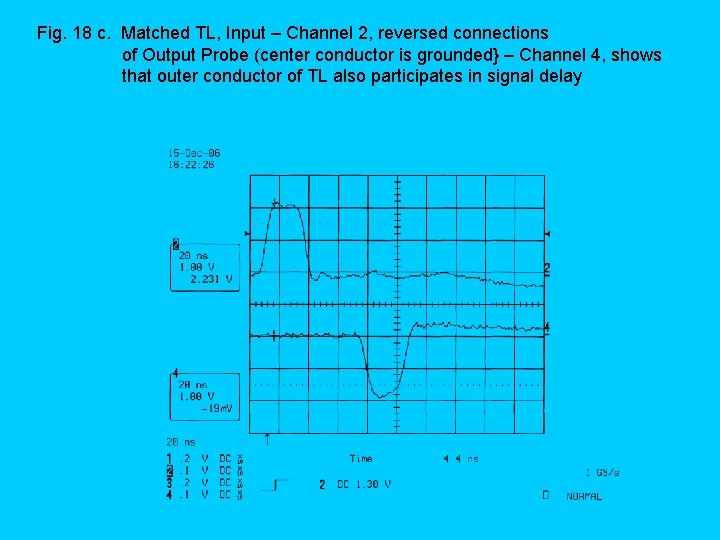
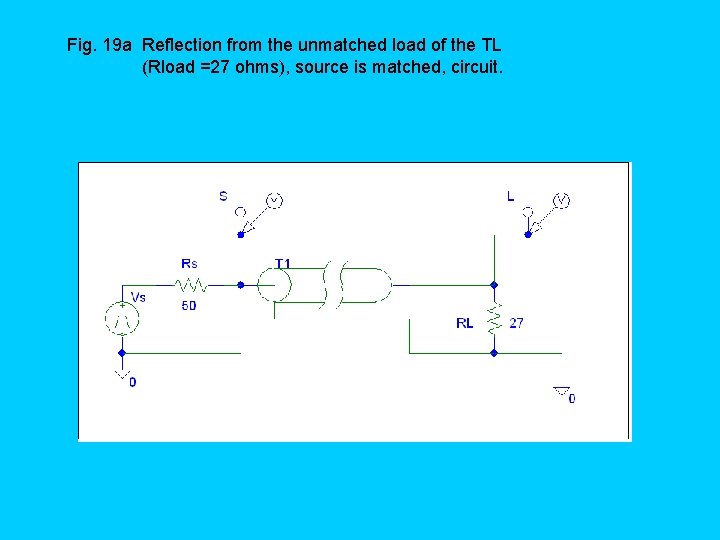
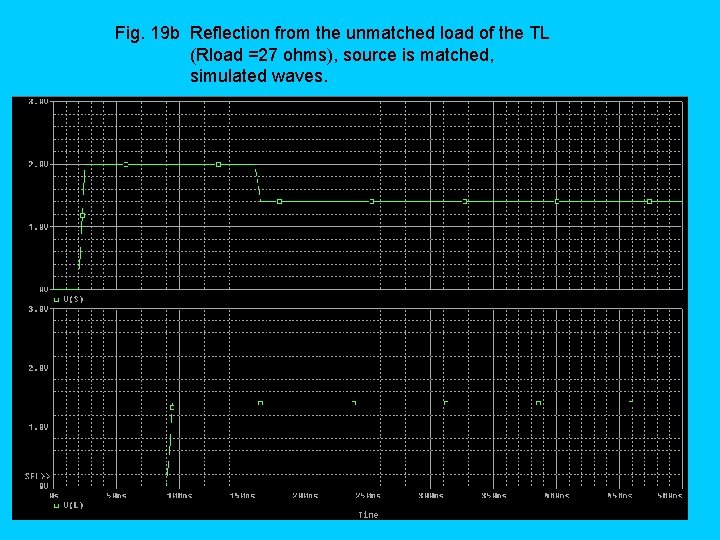
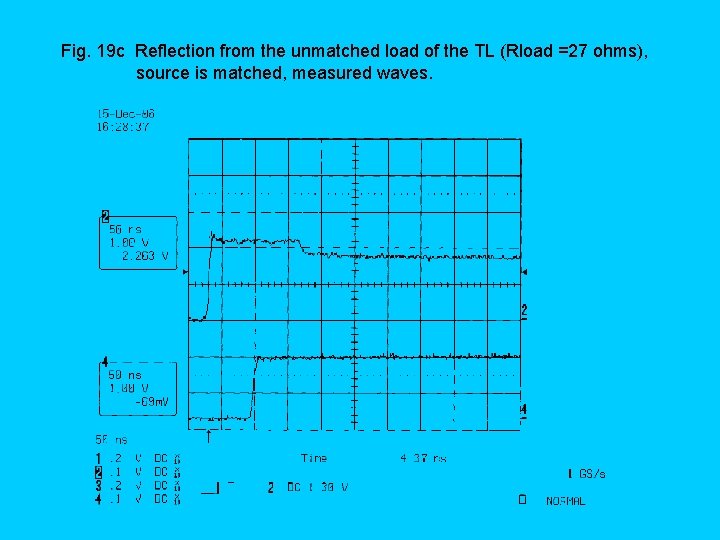
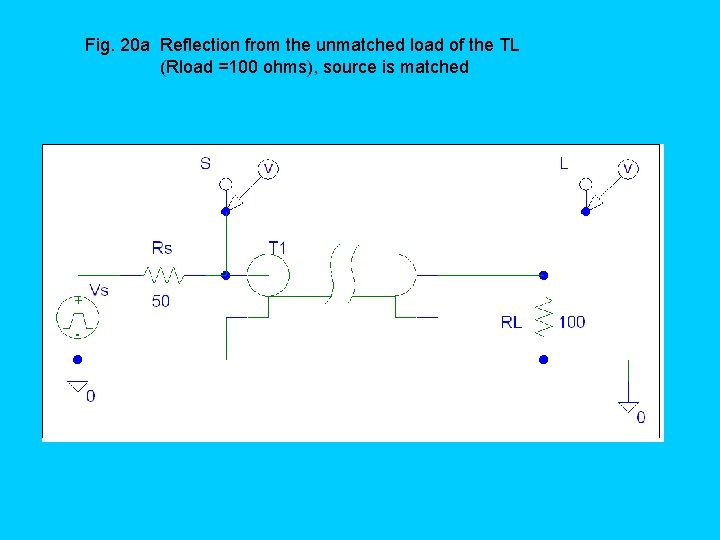
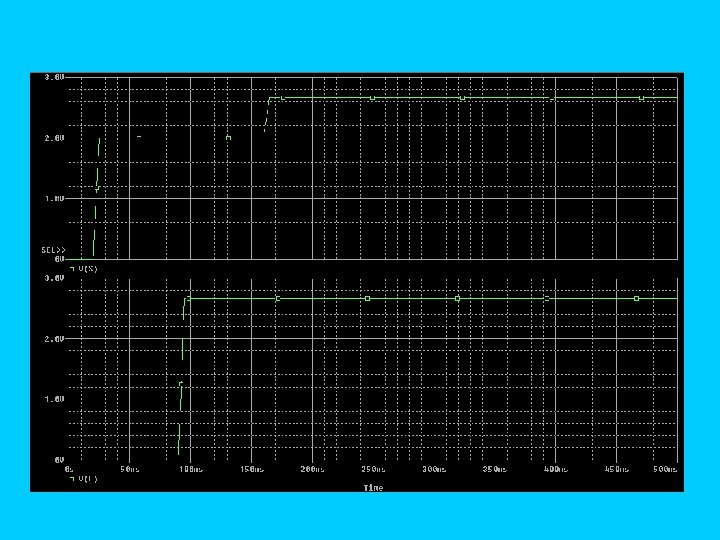
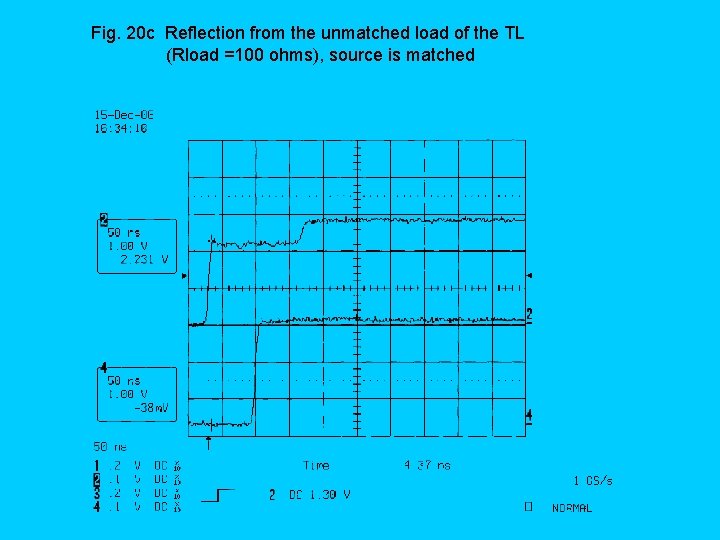
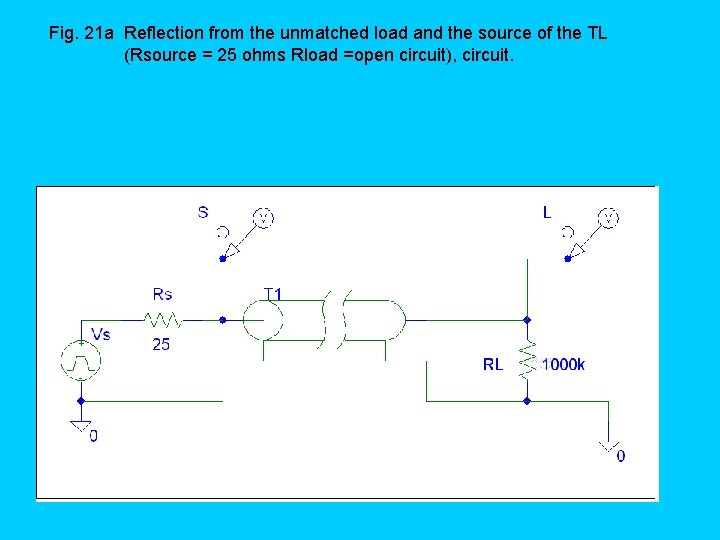
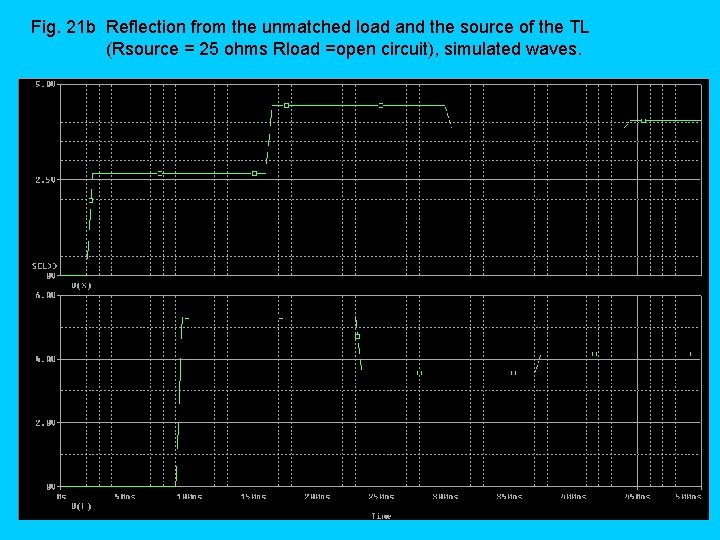
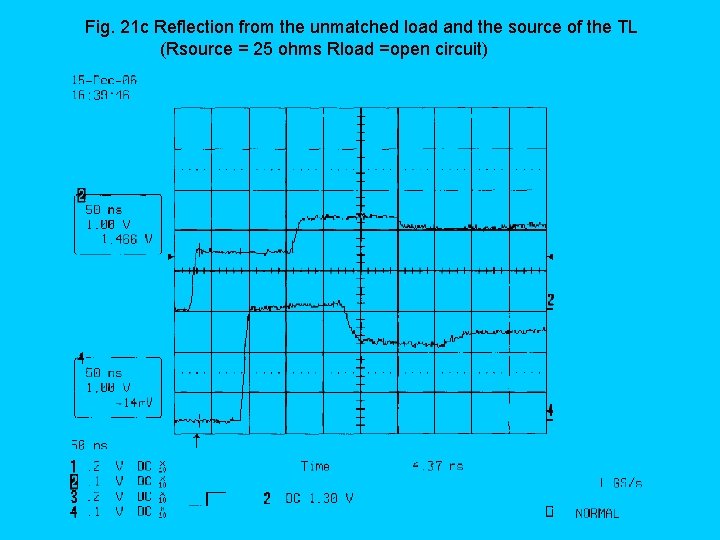
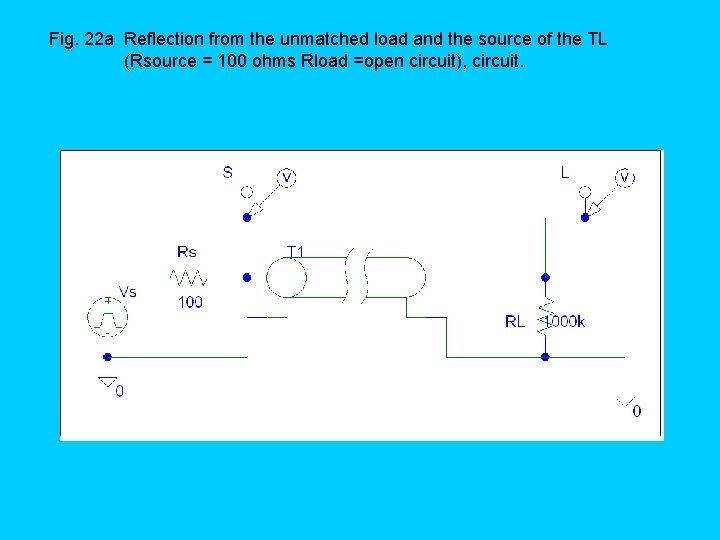
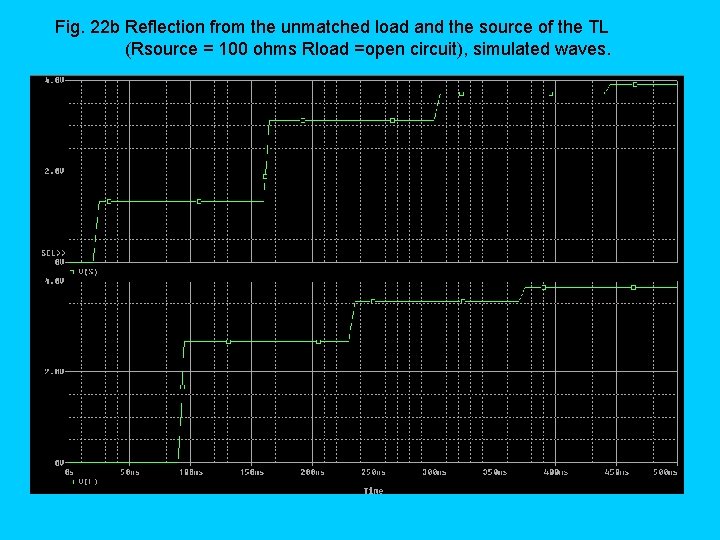
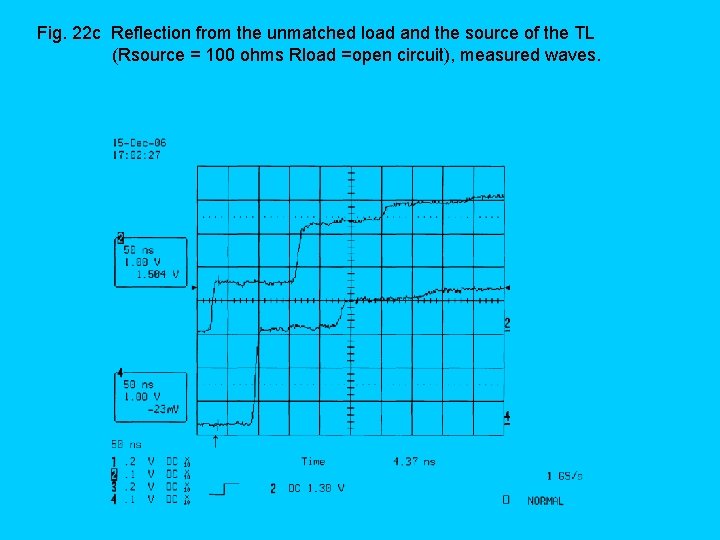
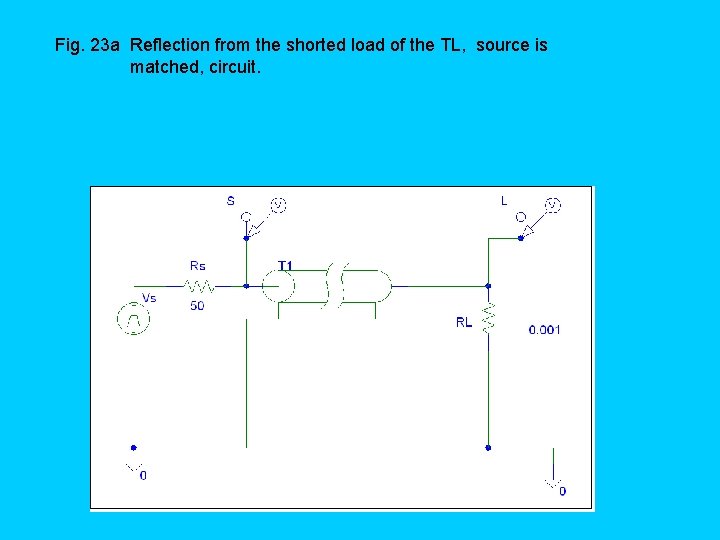
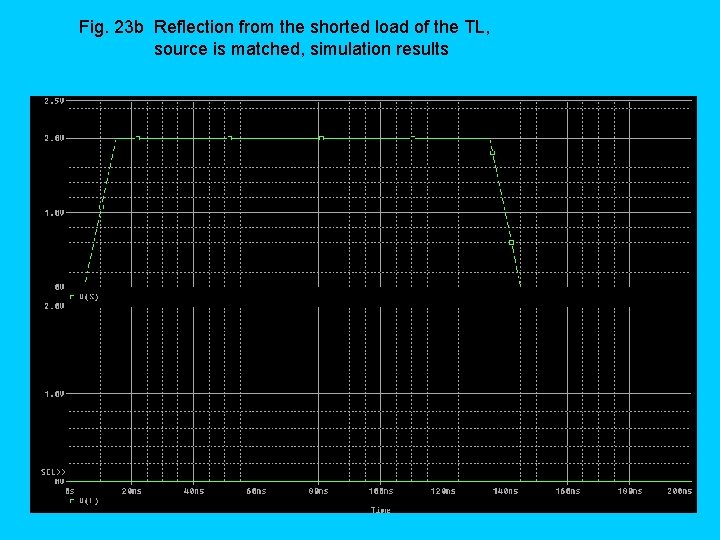
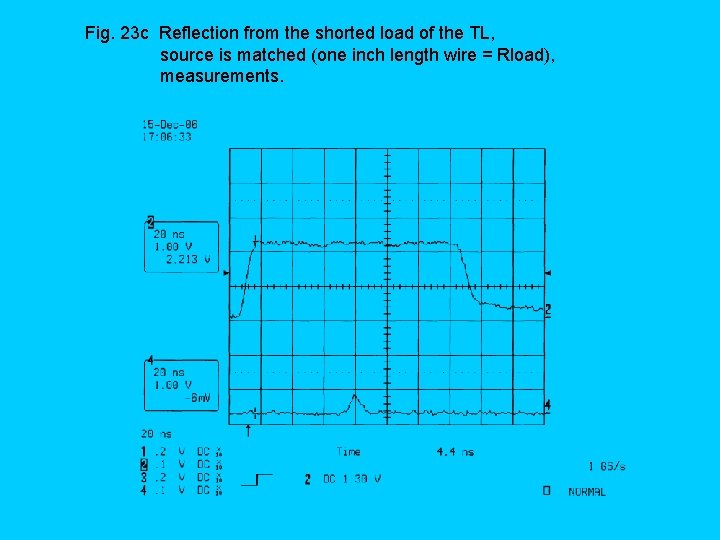
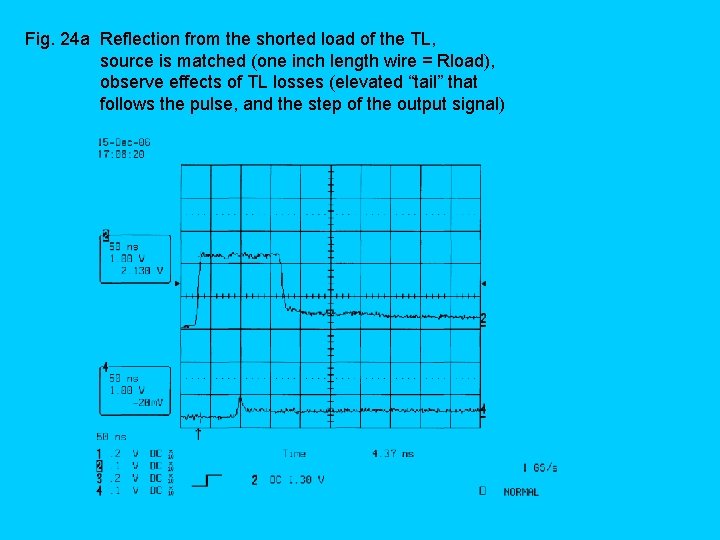
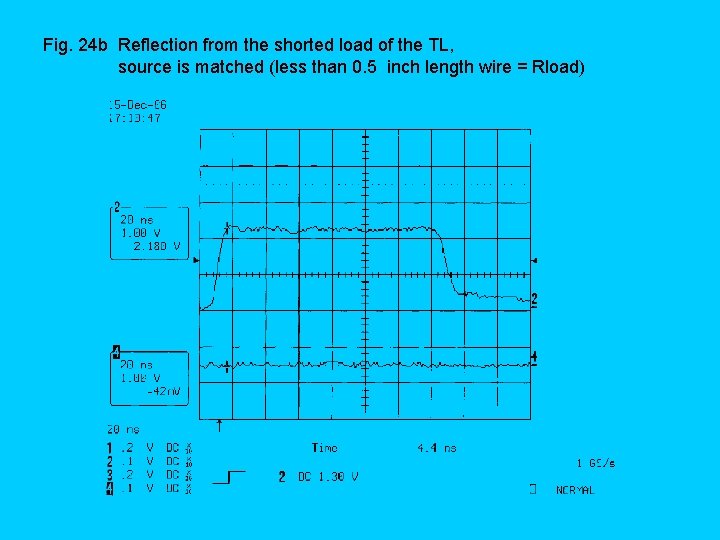
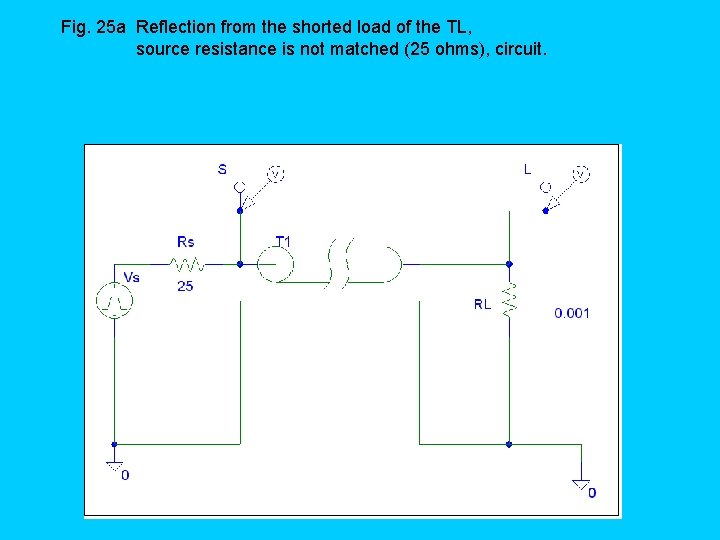
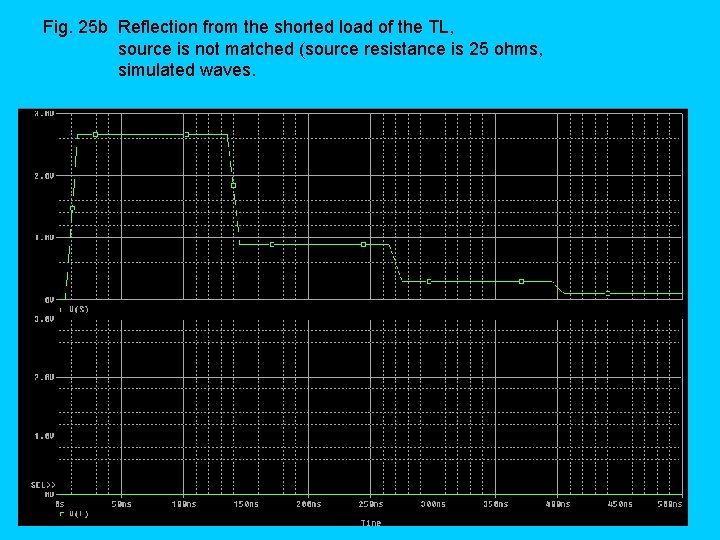
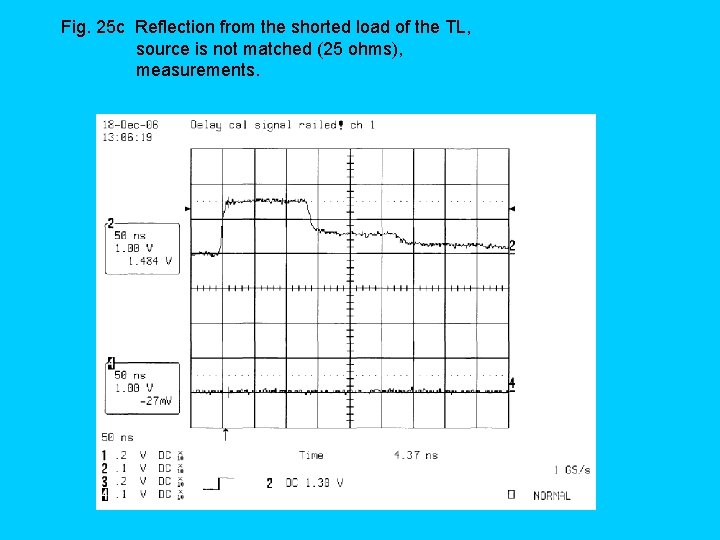
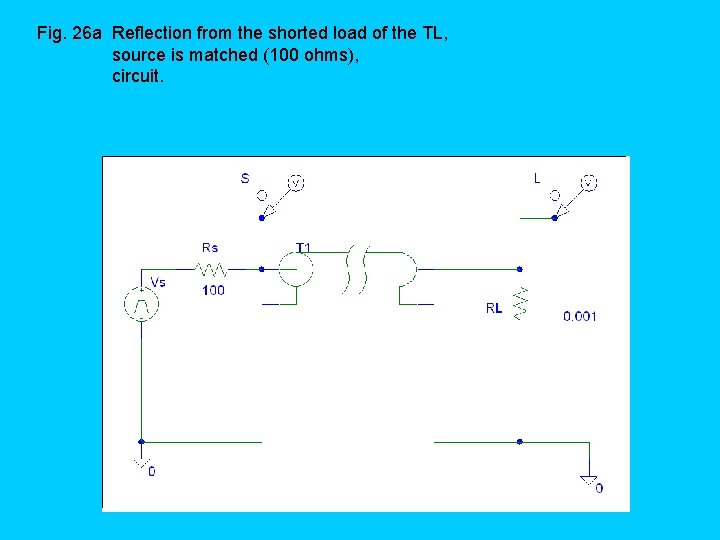
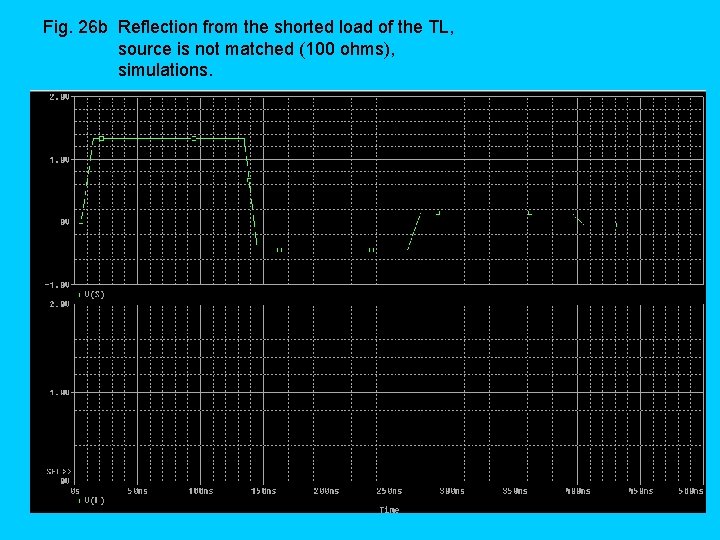
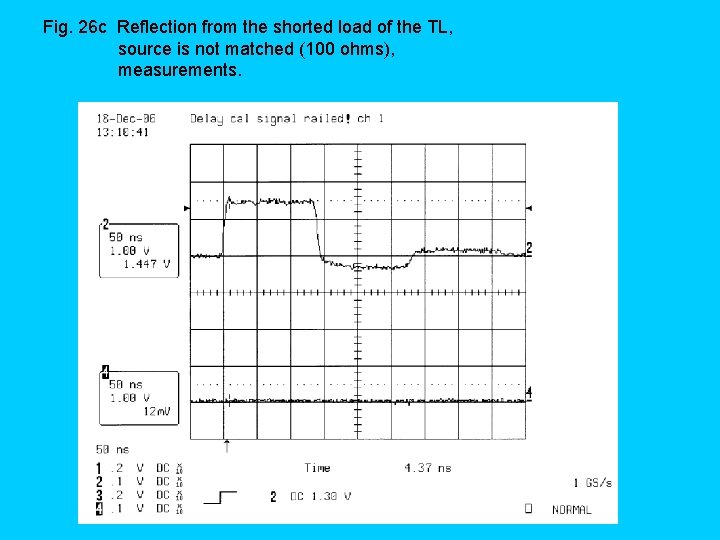
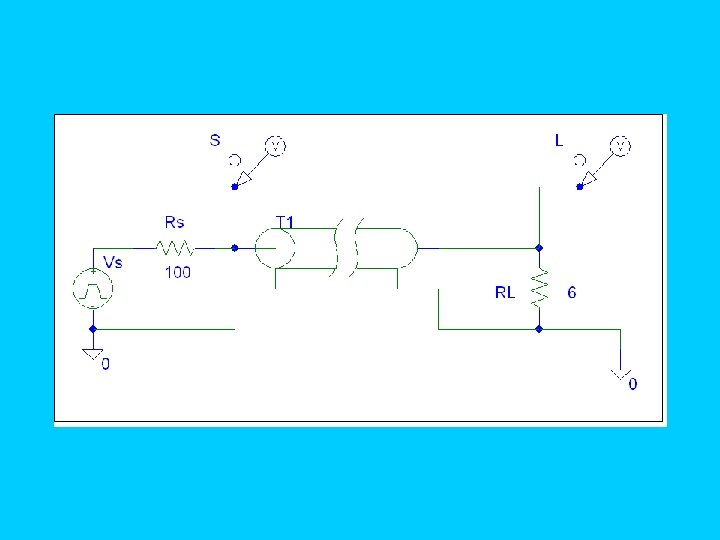
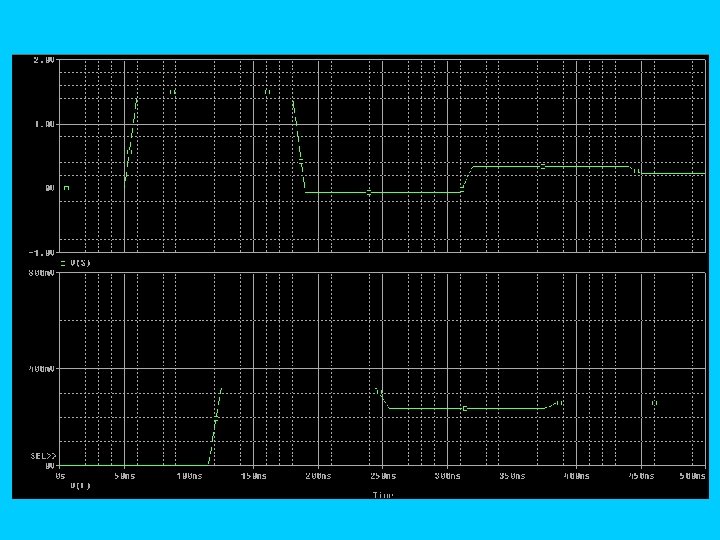
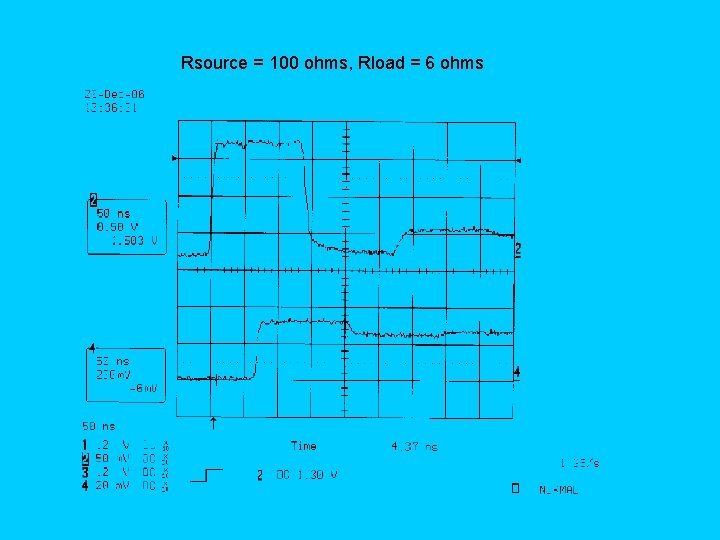
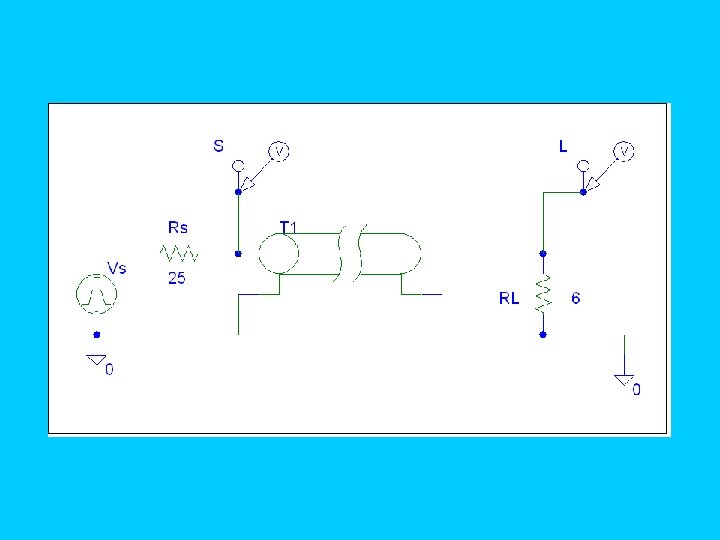
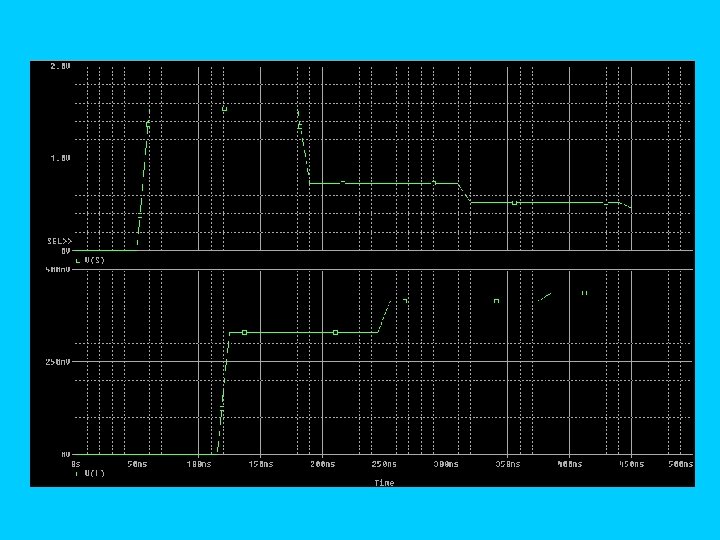
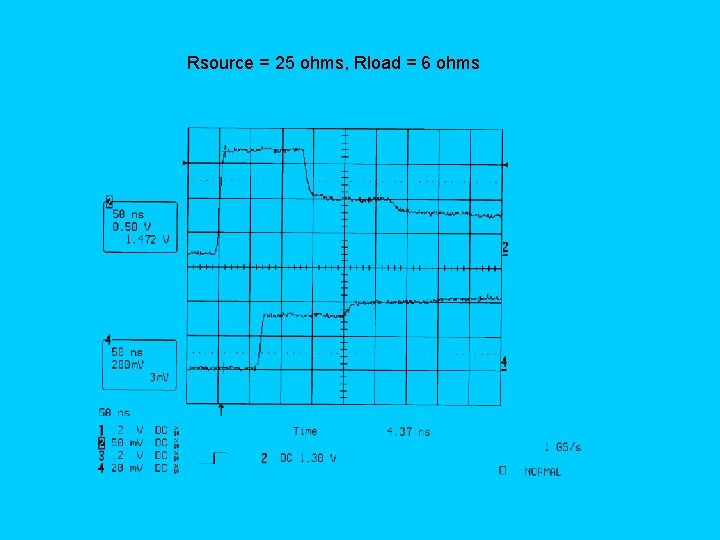
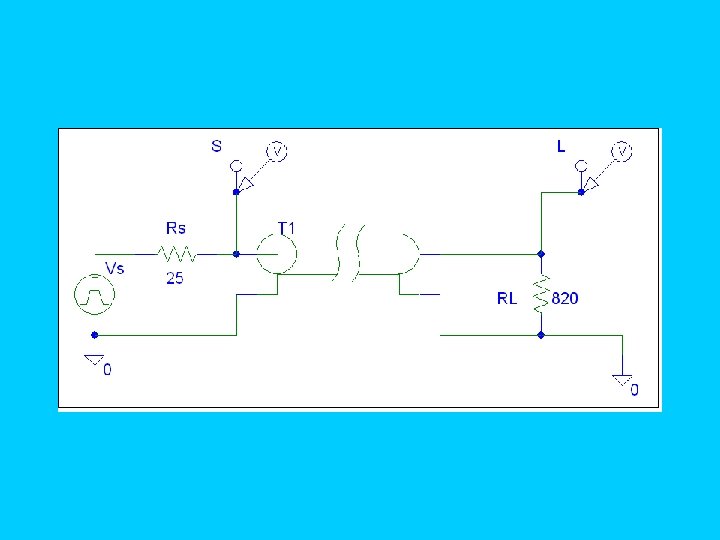
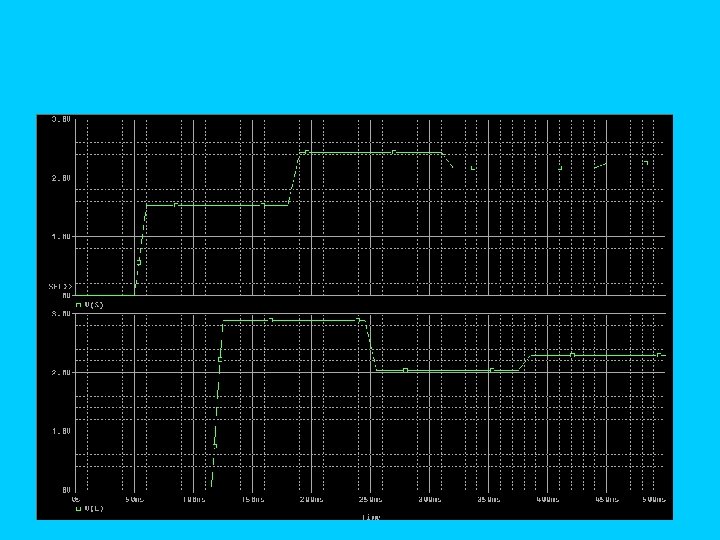
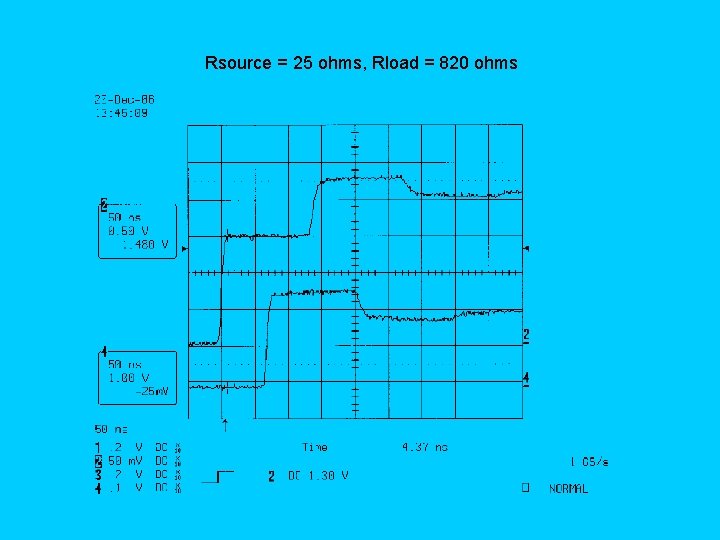
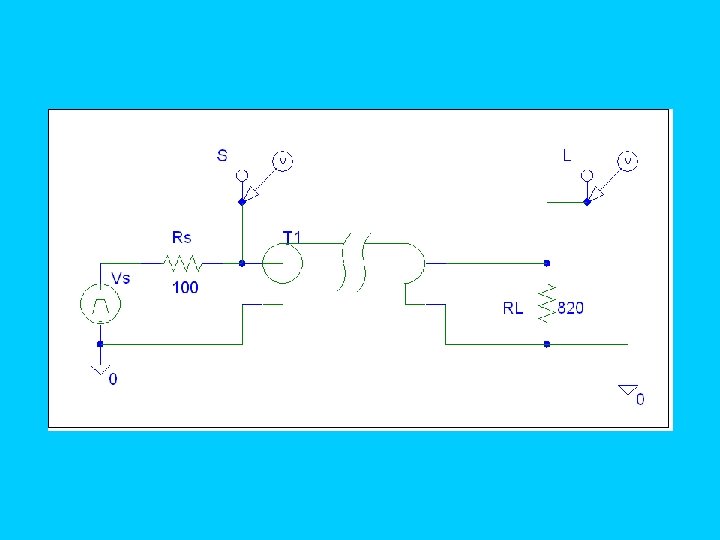
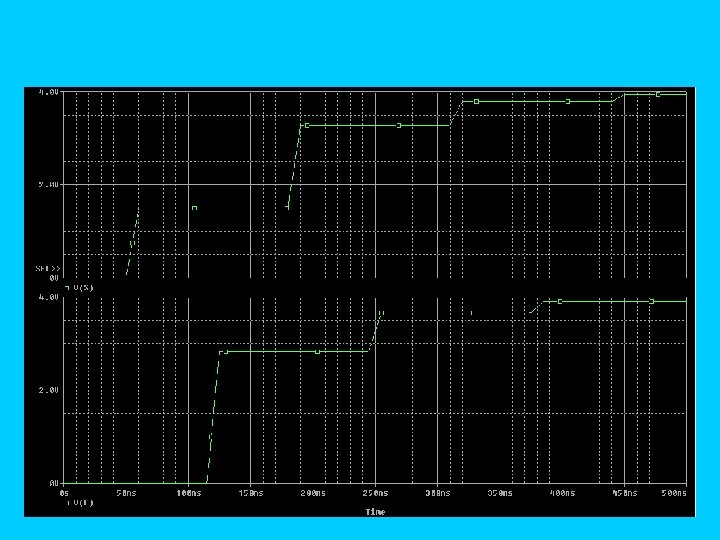
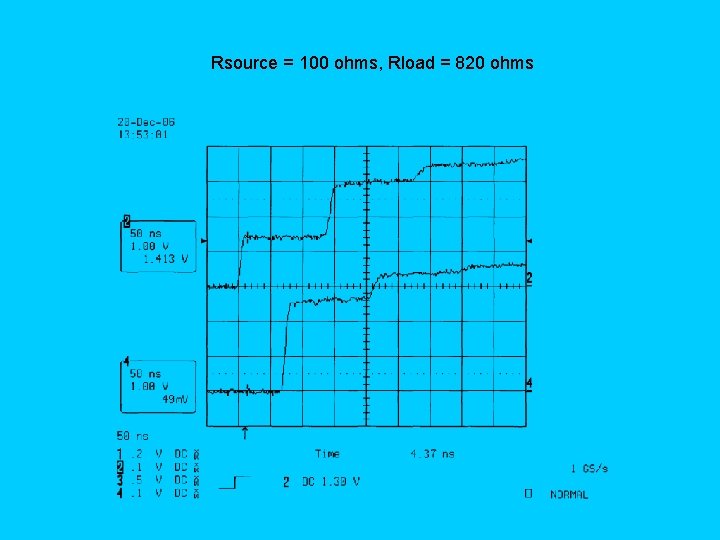
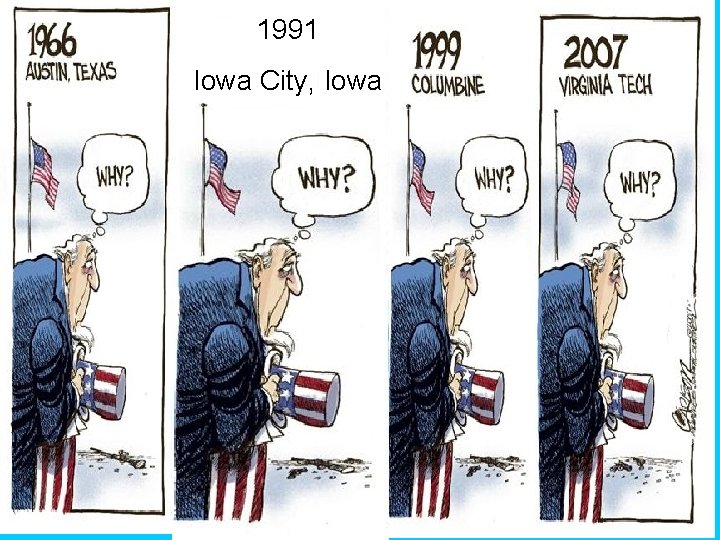
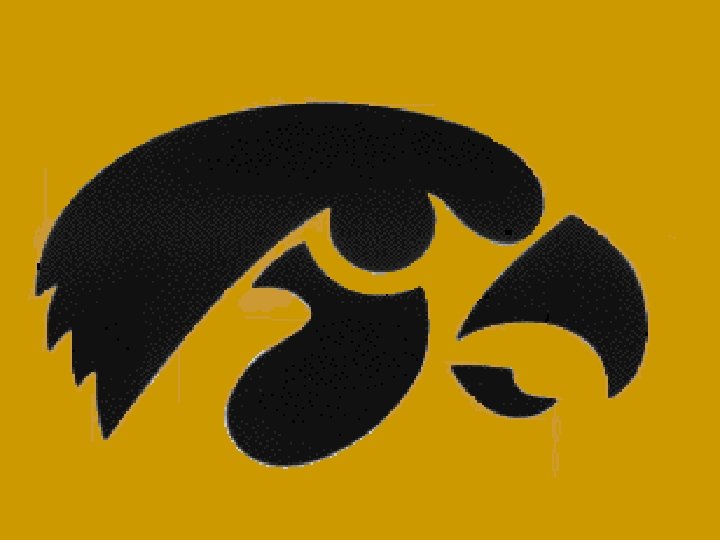
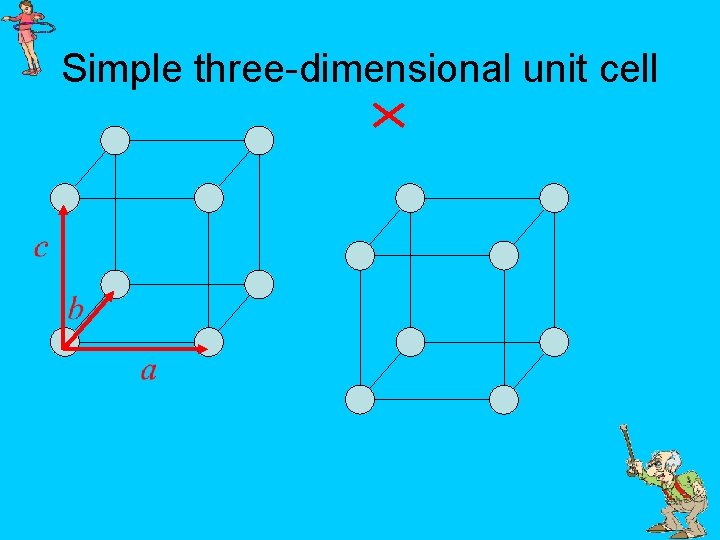
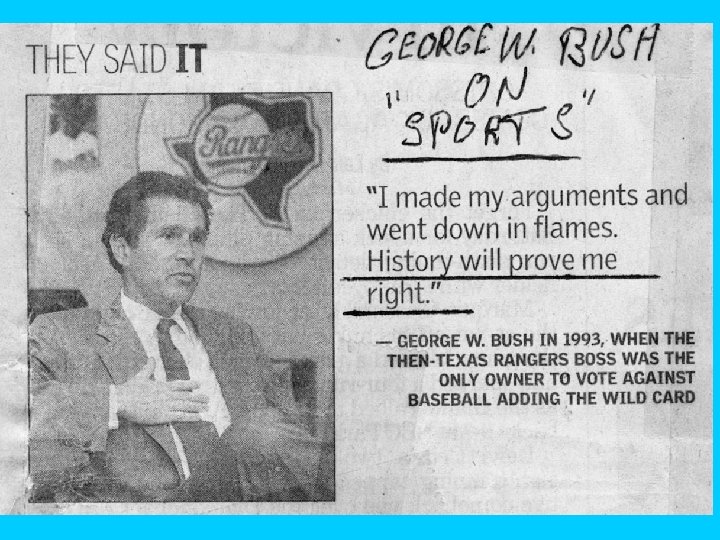
- Slides: 101
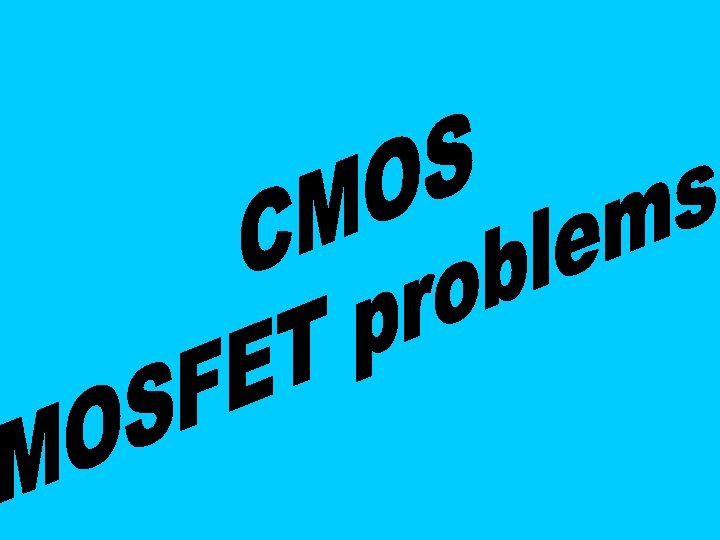
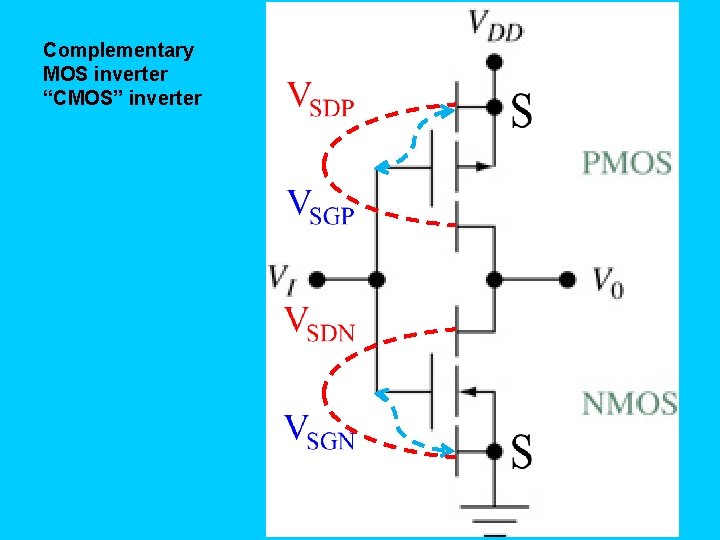
Complementary MOS inverter “CMOS” inverter
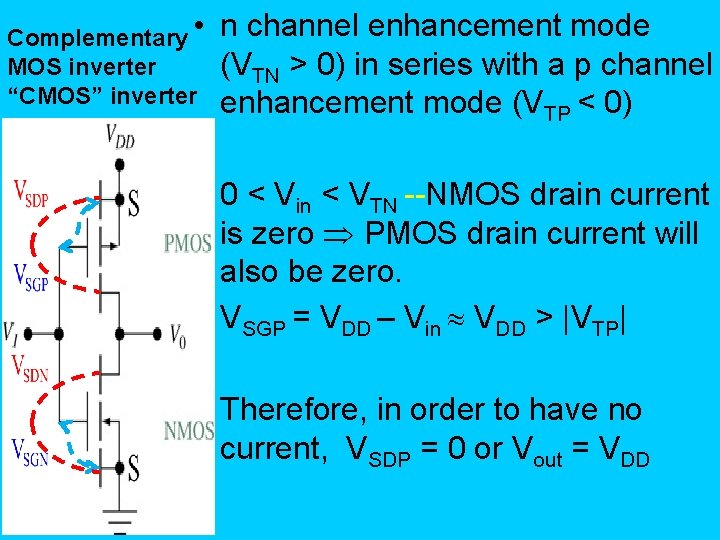
Complementary • MOS inverter “CMOS” inverter n channel enhancement mode (VTN > 0) in series with a p channel enhancement mode (VTP < 0) • 0 < Vin < VTN --NMOS drain current is zero PMOS drain current will also be zero. • VSGP = VDD – Vin VDD > |VTP| • Therefore, in order to have no current, VSDP = 0 or Vout = VDD
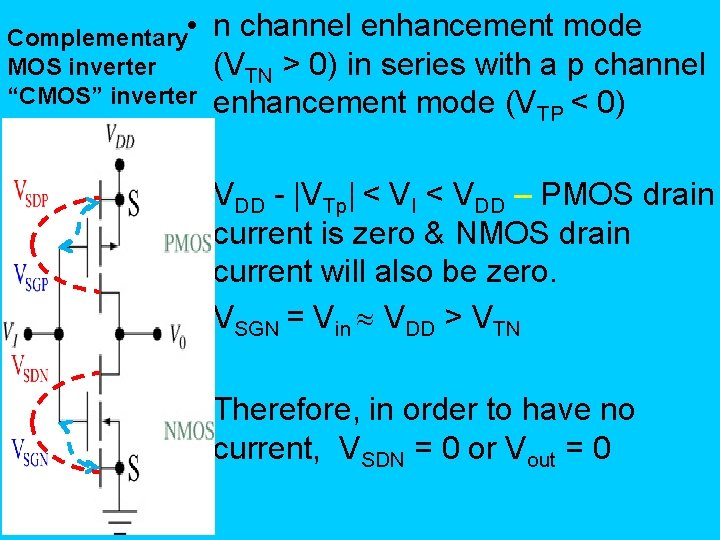
Complementary • MOS inverter “CMOS” inverter n channel enhancement mode (VTN > 0) in series with a p channel enhancement mode (VTP < 0) • VDD - |VTp| < VI < VDD – PMOS drain current is zero & NMOS drain current will also be zero. • VSGN = Vin VDD > VTN • Therefore, in order to have no current, VSDN = 0 or Vout = 0
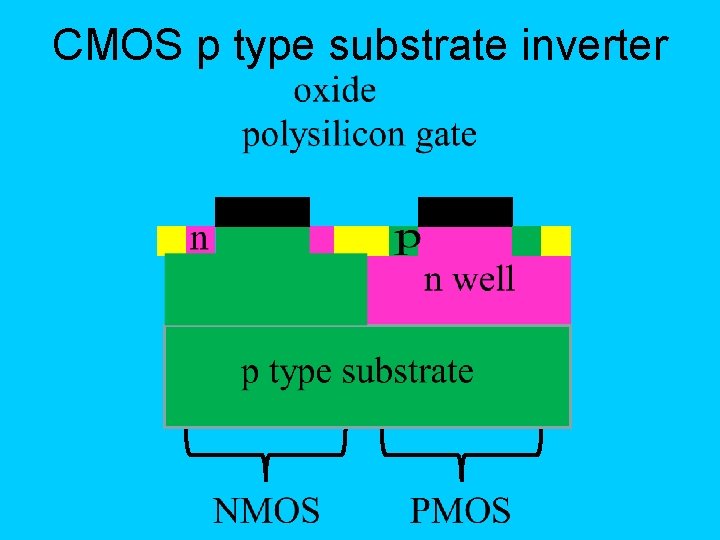
CMOS p type substrate inverter
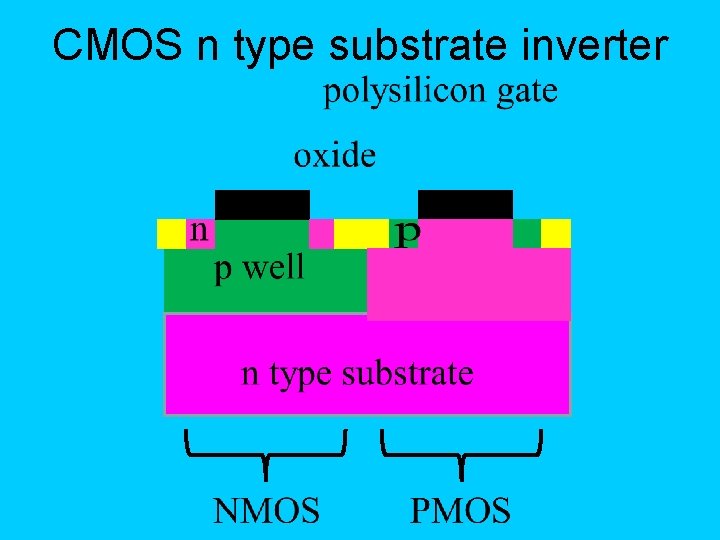
CMOS n type substrate inverter
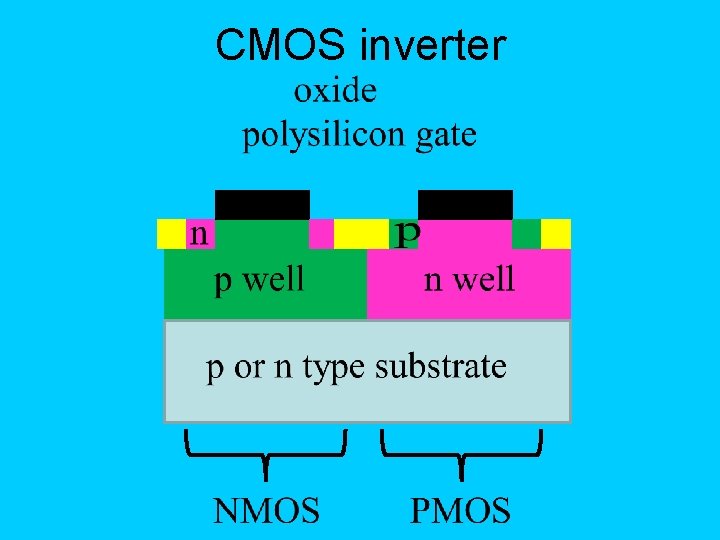
CMOS inverter
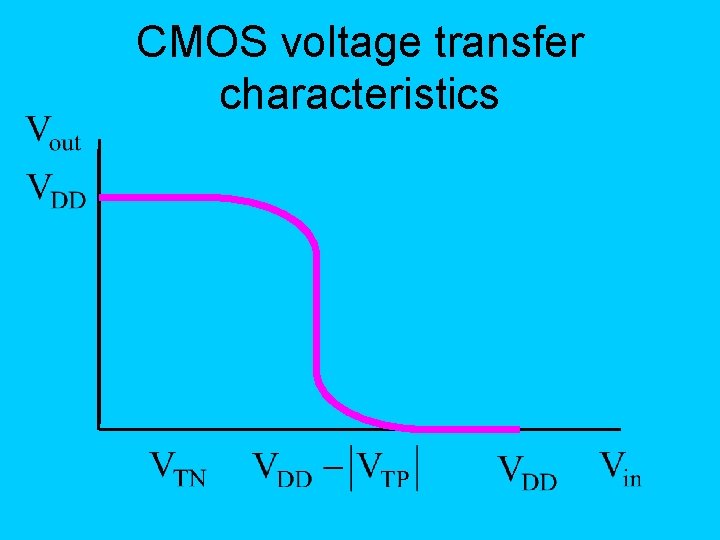
CMOS voltage transfer characteristics
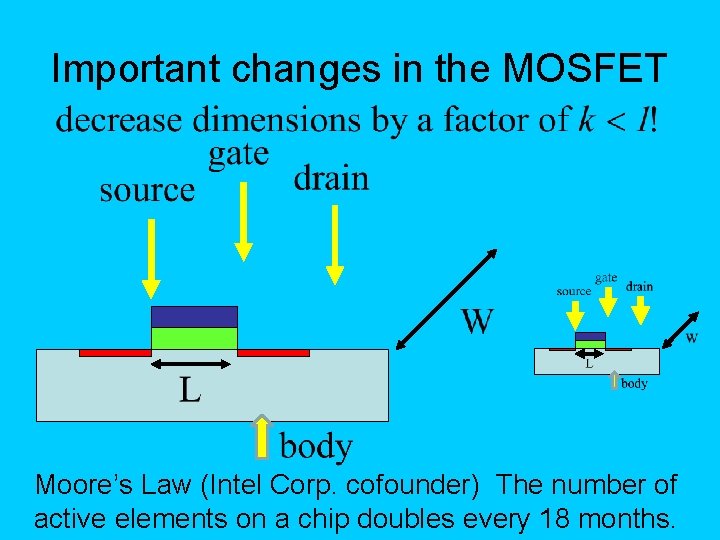
Important changes in the MOSFET Moore’s Law (Intel Corp. cofounder) The number of active elements on a chip doubles every 18 months.
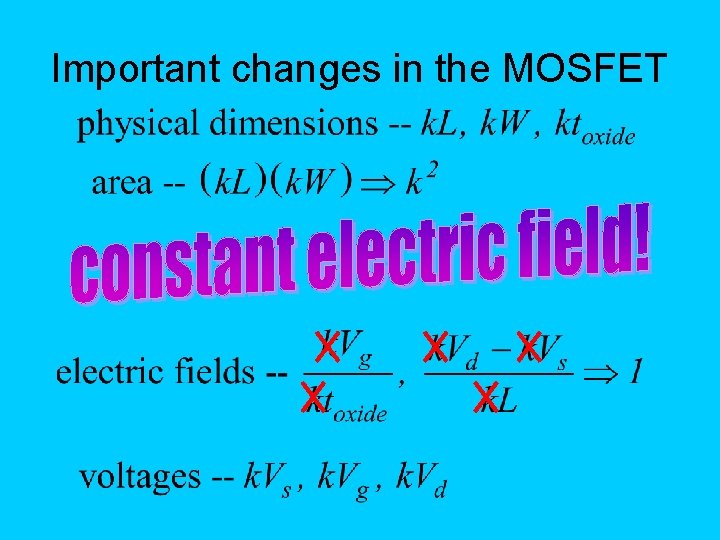
Important changes in the MOSFET
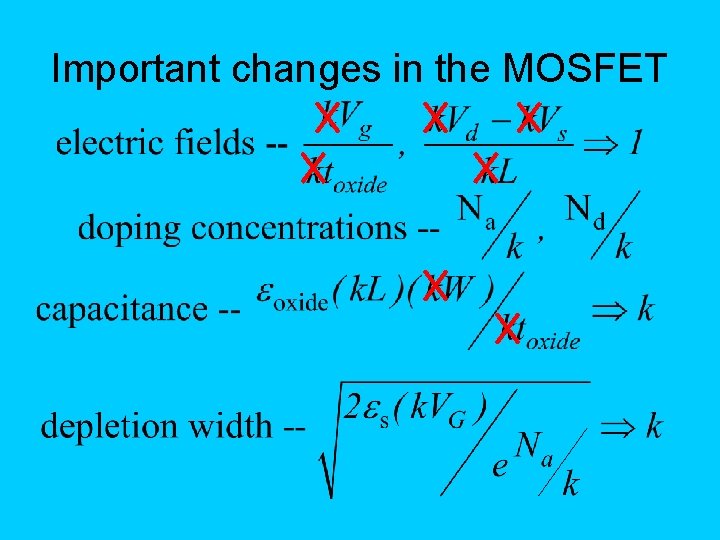
Important changes in the MOSFET
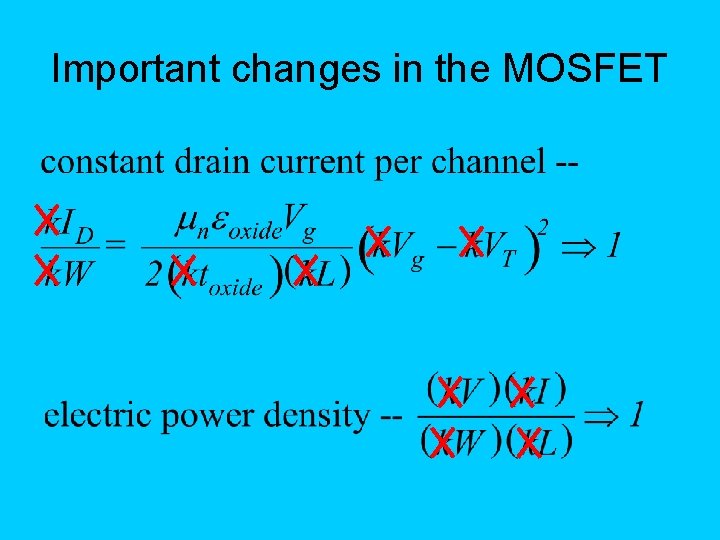
Important changes in the MOSFET
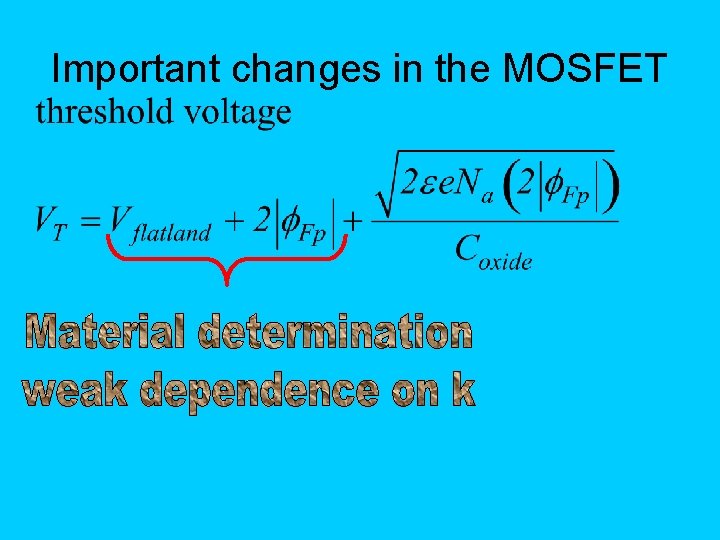
Important changes in the MOSFET
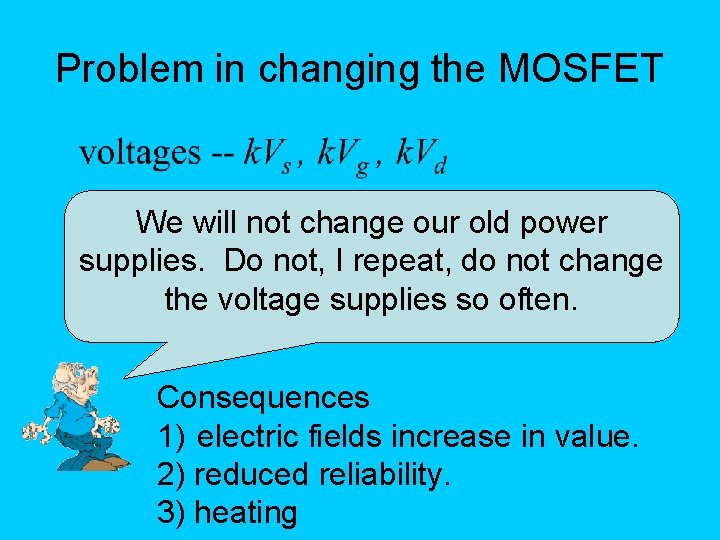
Problem in changing the MOSFET We will not change our old power supplies. Do not, I repeat, do not change the voltage supplies so often. Consequences 1) electric fields increase in value. 2) reduced reliability. 3) heating
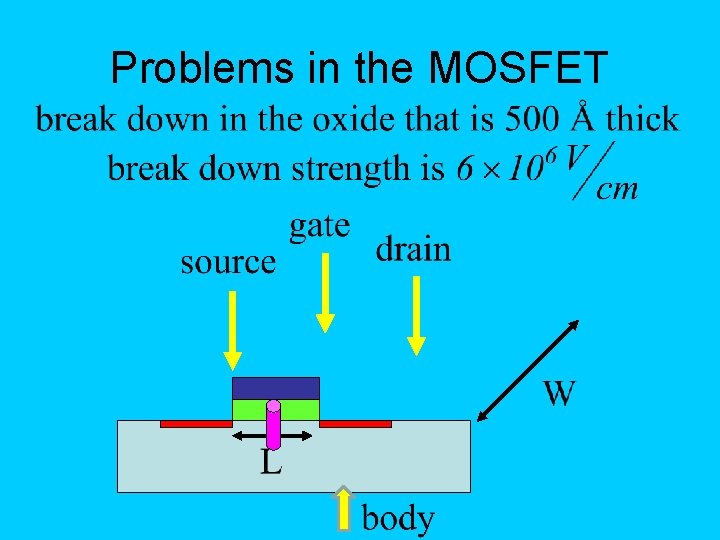
Problems in the MOSFET
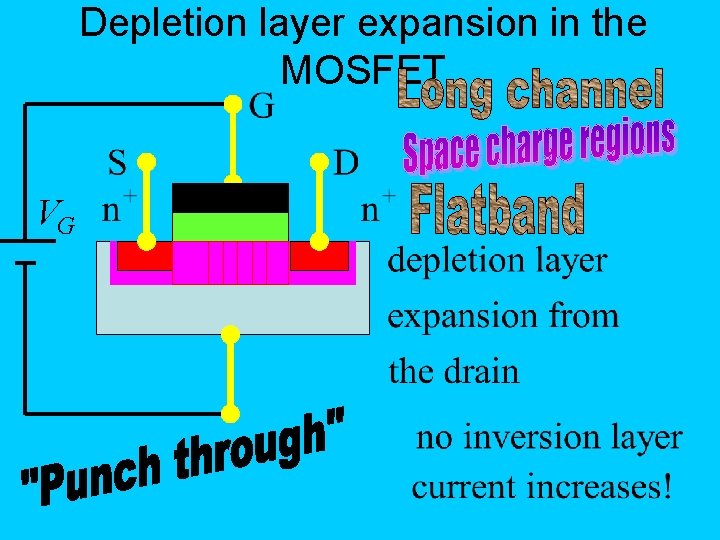
Depletion layer expansion in the MOSFET VG
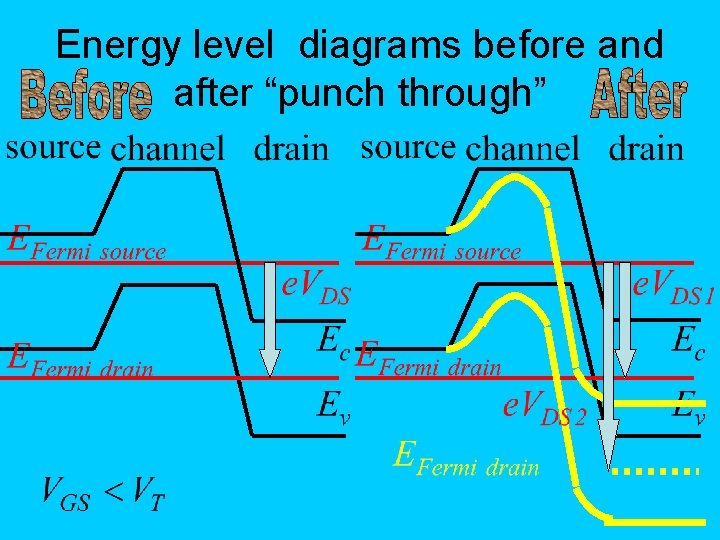
Energy level diagrams before and after “punch through”
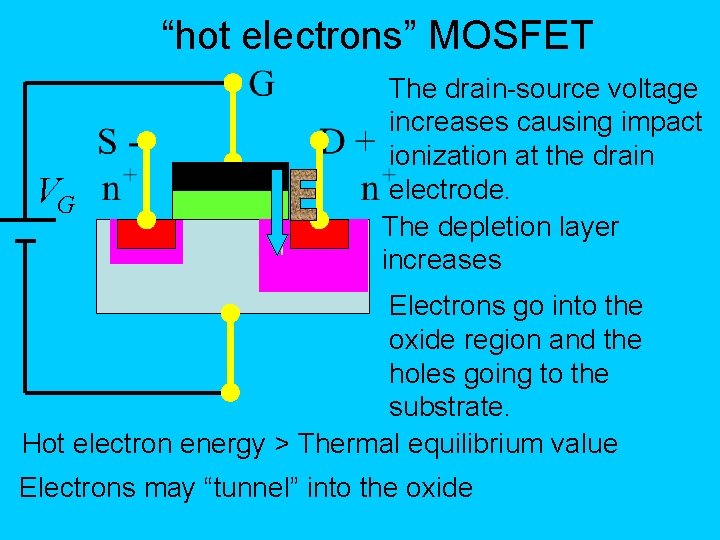
“hot electrons” MOSFET VG The drain-source voltage increases causing impact ionization at the drain electrode. The depletion layer increases Electrons go into the oxide region and the holes going to the substrate. Hot electron energy > Thermal equilibrium value Electrons may “tunnel” into the oxide
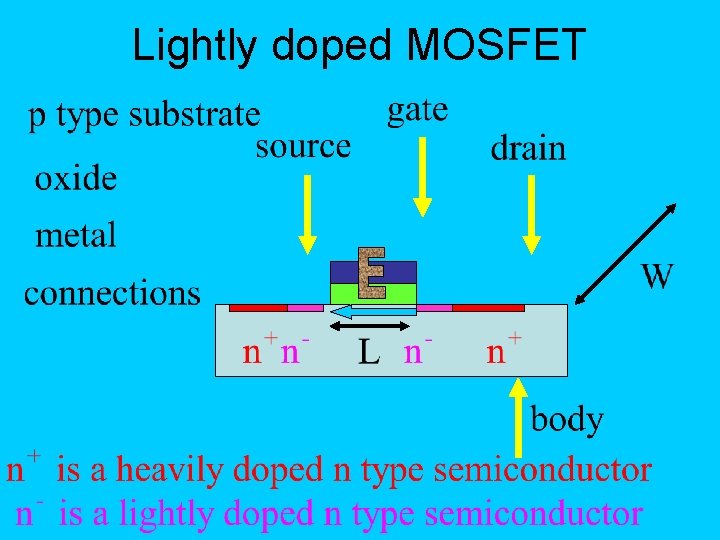
Lightly doped MOSFET
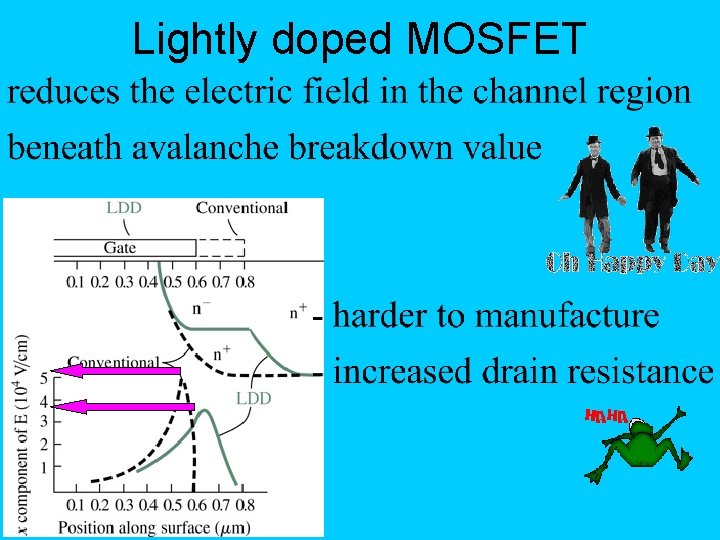
Lightly doped MOSFET
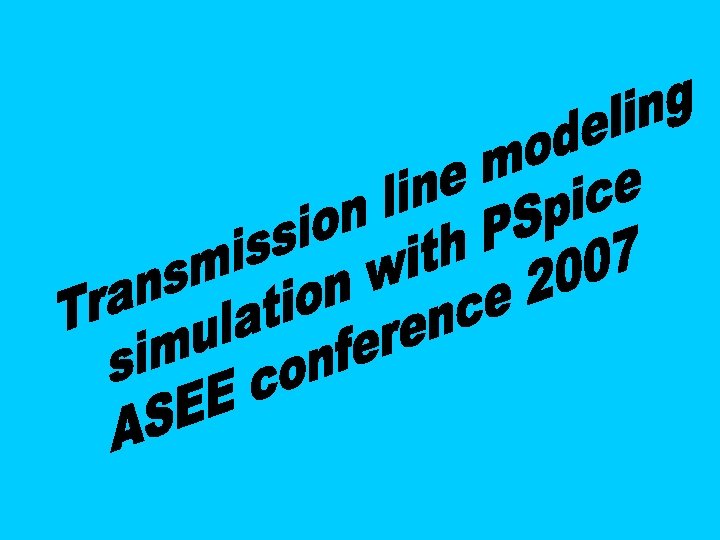
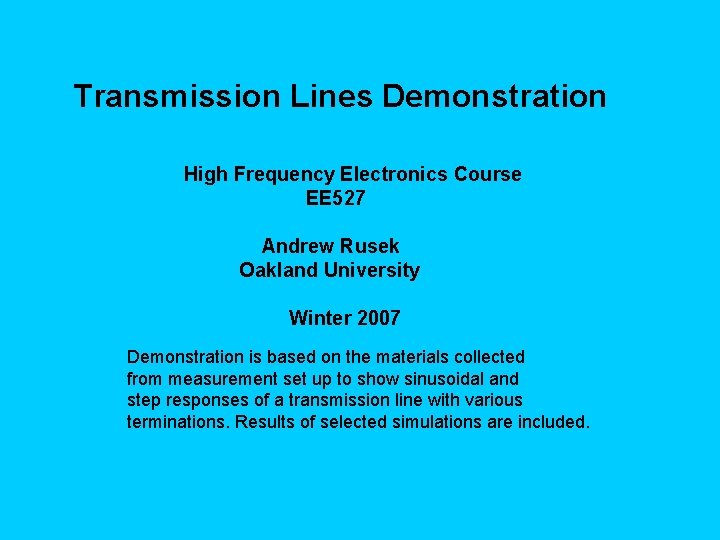
Transmission Lines Demonstration High Frequency Electronics Course EE 527 Andrew Rusek Oakland University Winter 2007 Demonstration is based on the materials collected from measurement set up to show sinusoidal and step responses of a transmission line with various terminations. Results of selected simulations are included.
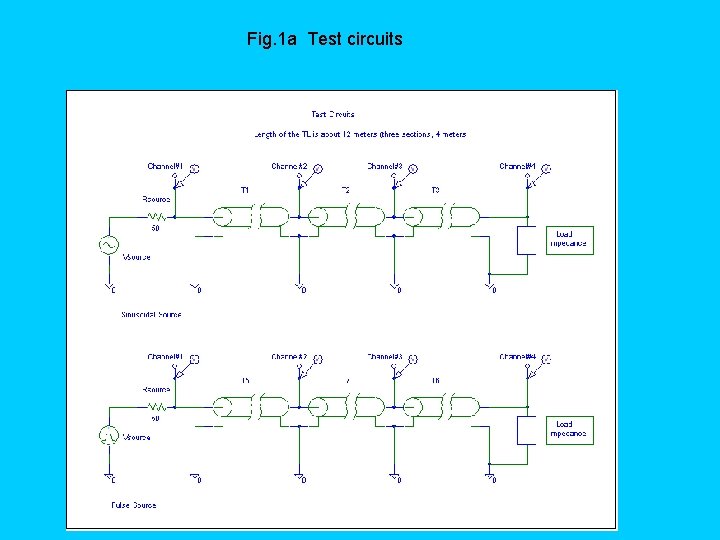
Fig. 1 a Test circuits
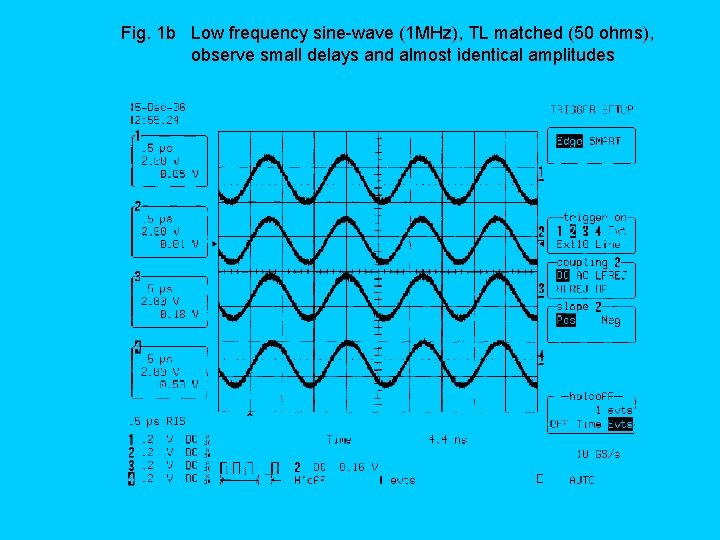
Fig. 1 b Low frequency sine-wave (1 MHz), TL matched (50 ohms), observe small delays and almost identical amplitudes
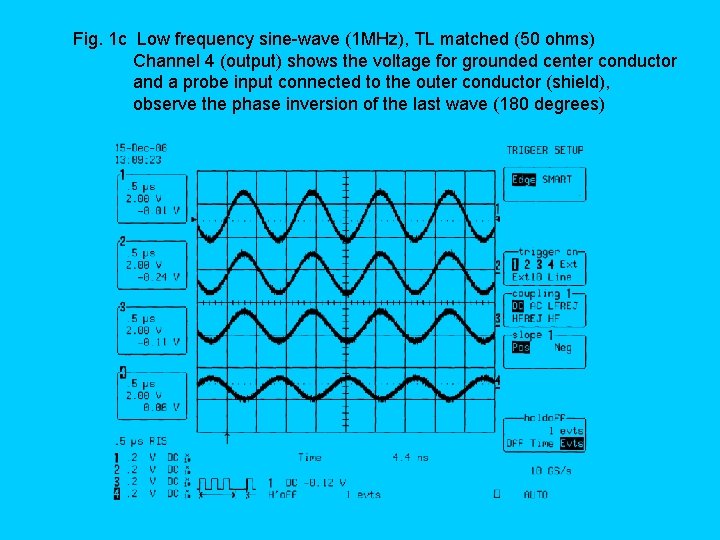
Fig. 1 c Low frequency sine-wave (1 MHz), TL matched (50 ohms) Channel 4 (output) shows the voltage for grounded center conductor and a probe input connected to the outer conductor (shield), observe the phase inversion of the last wave (180 degrees)
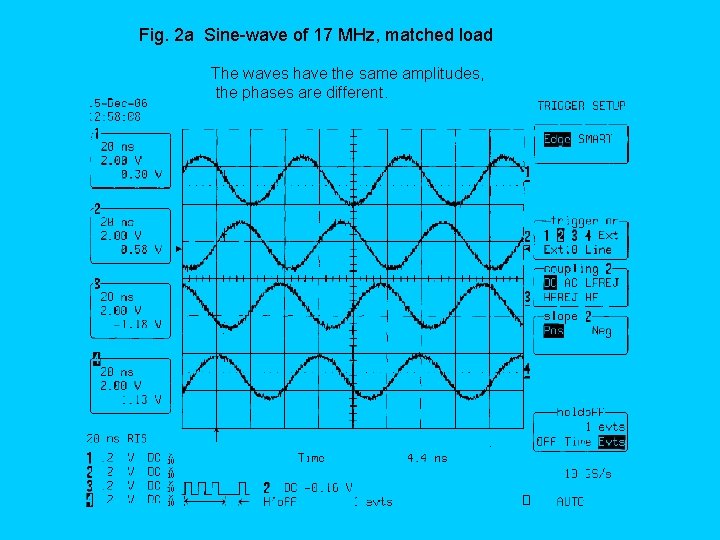
Fig. 2 a Sine-wave of 17 MHz, matched load The waves have the same amplitudes, the phases are different.
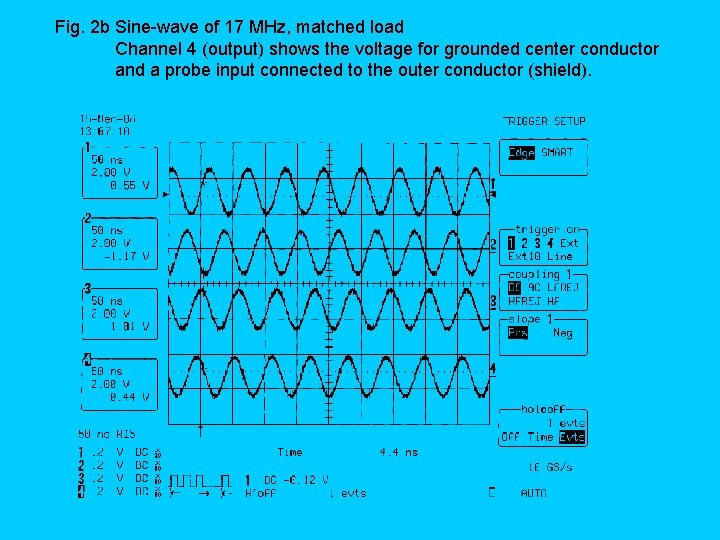
Fig. 2 b Sine-wave of 17 MHz, matched load Channel 4 (output) shows the voltage for grounded center conductor and a probe input connected to the outer conductor (shield).
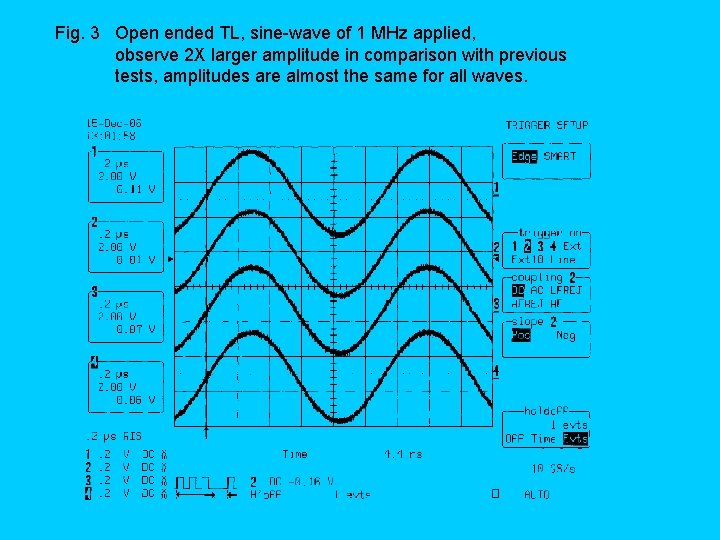
Fig. 3 Open ended TL, sine-wave of 1 MHz applied, observe 2 X larger amplitude in comparison with previous tests, amplitudes are almost the same for all waves.
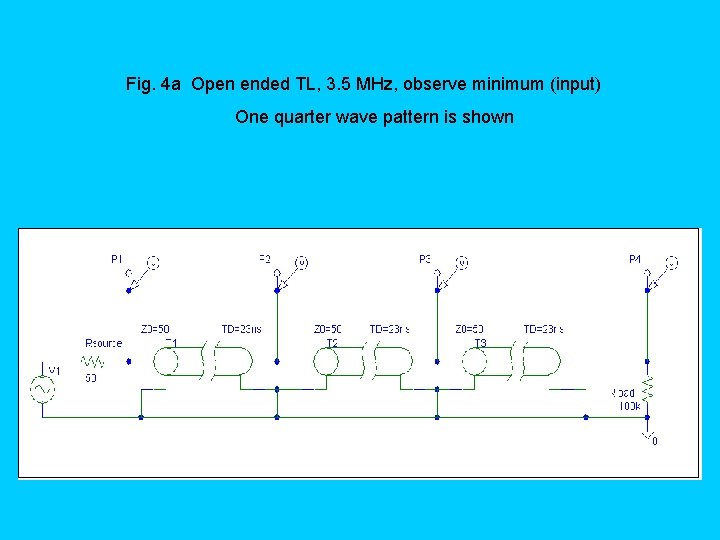
Fig. 4 a Open ended TL, 3. 5 MHz, observe minimum (input) One quarter wave pattern is shown
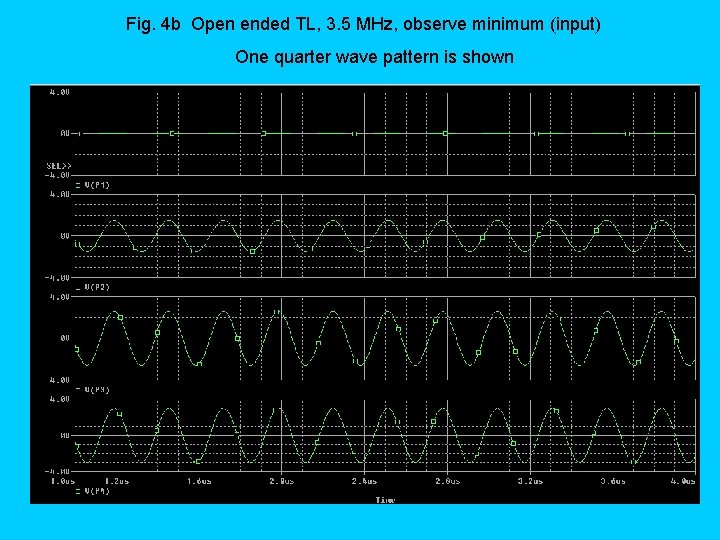
Fig. 4 b Open ended TL, 3. 5 MHz, observe minimum (input) One quarter wave pattern is shown
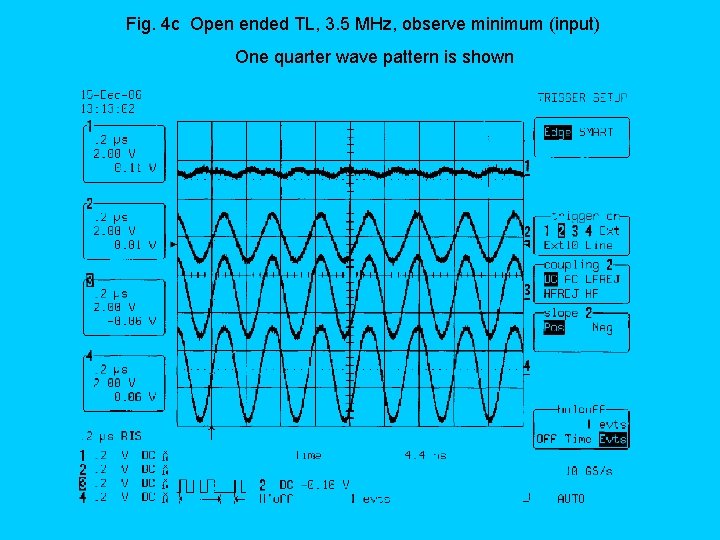
Fig. 4 c Open ended TL, 3. 5 MHz, observe minimum (input) One quarter wave pattern is shown
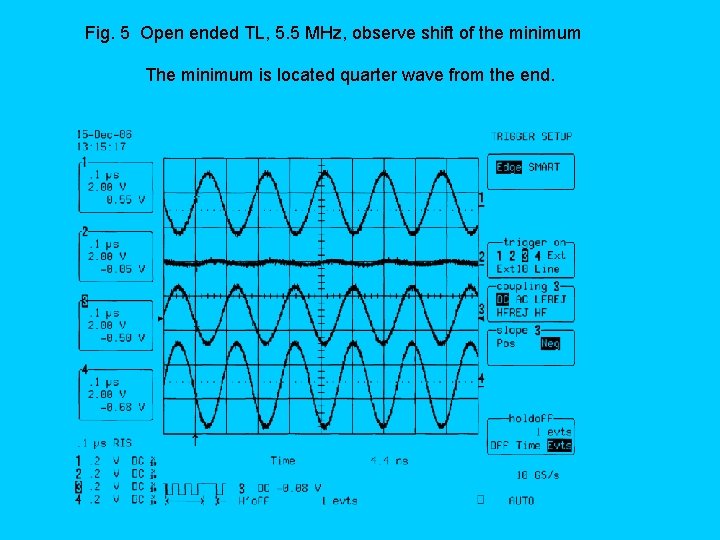
Fig. 5 Open ended TL, 5. 5 MHz, observe shift of the minimum The minimum is located quarter wave from the end.
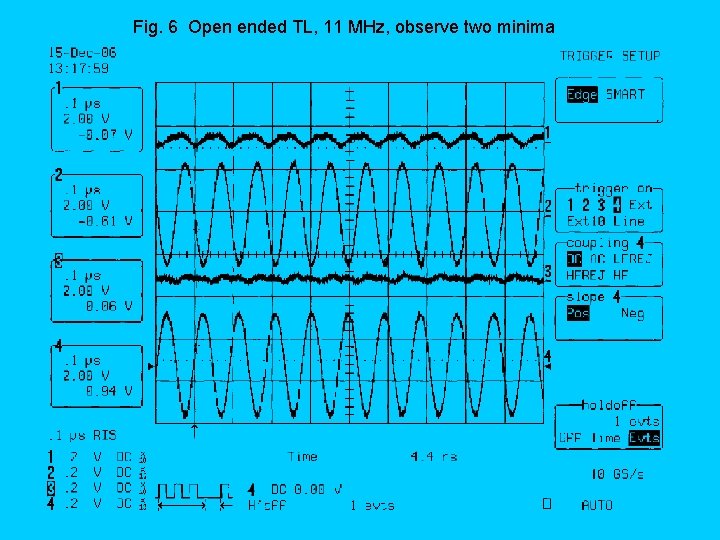
Fig. 6 Open ended TL, 11 MHz, observe two minima
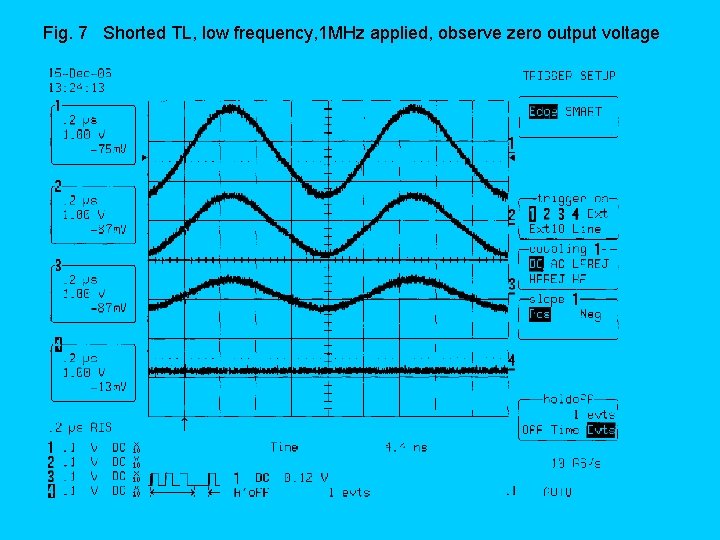
Fig. 7 Shorted TL, low frequency, 1 MHz applied, observe zero output voltage
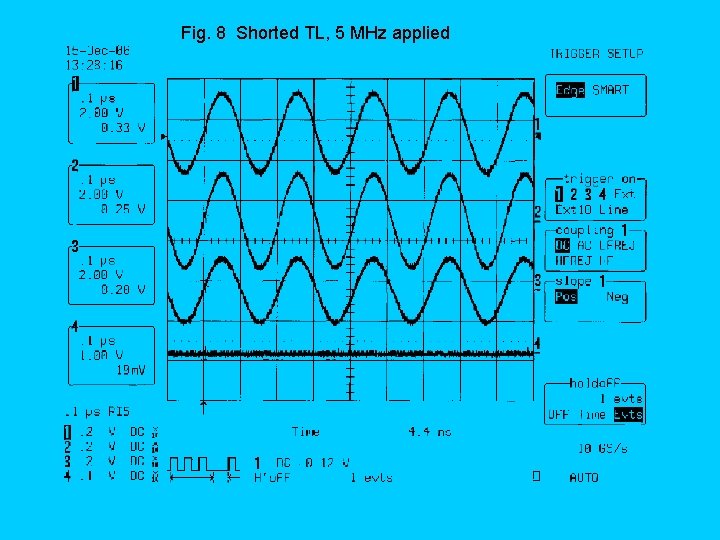
Fig. 8 Shorted TL, 5 MHz applied
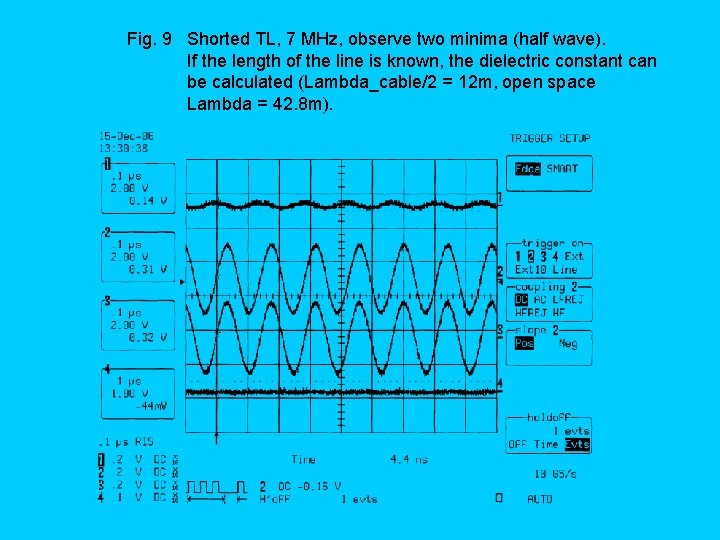
Fig. 9 Shorted TL, 7 MHz, observe two minima (half wave). If the length of the line is known, the dielectric constant can be calculated (Lambda_cable/2 = 12 m, open space Lambda = 42. 8 m).
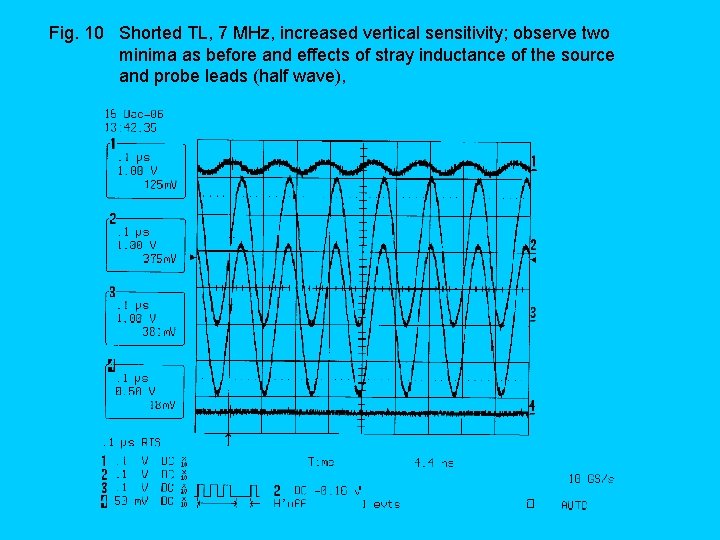
Fig. 10 Shorted TL, 7 MHz, increased vertical sensitivity; observe two minima as before and effects of stray inductance of the source and probe leads (half wave),
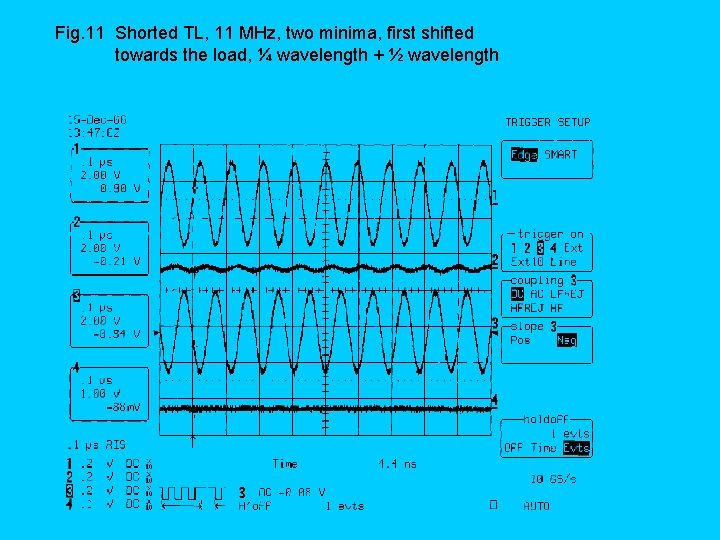
Fig. 11 Shorted TL, 11 MHz, two minima, first shifted towards the load, ¼ wavelength + ½ wavelength
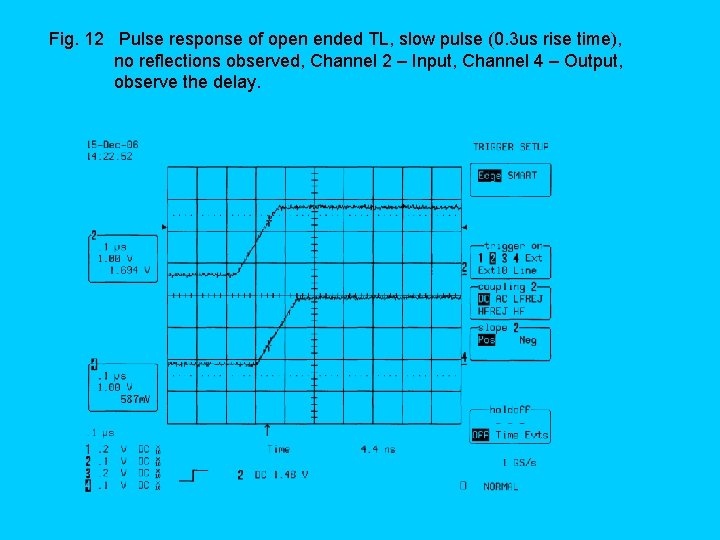
Fig. 12 Pulse response of open ended TL, slow pulse (0. 3 us rise time), no reflections observed, Channel 2 – Input, Channel 4 – Output, observe the delay.
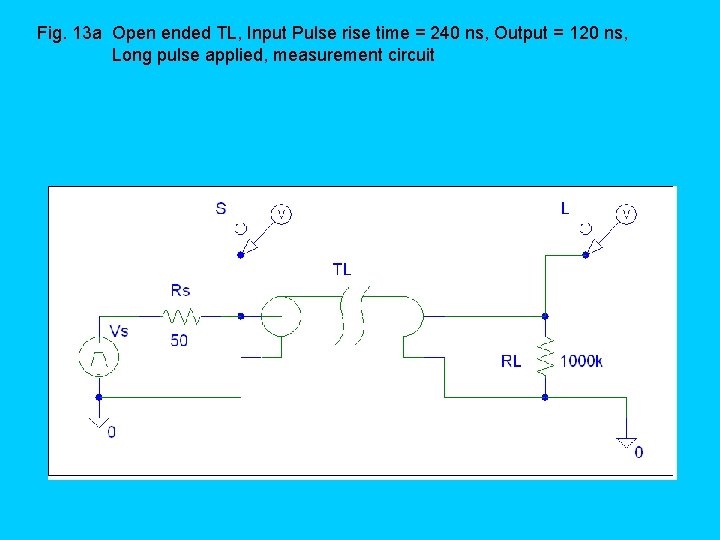
Fig. 13 a Open ended TL, Input Pulse rise time = 240 ns, Output = 120 ns, Long pulse applied, measurement circuit
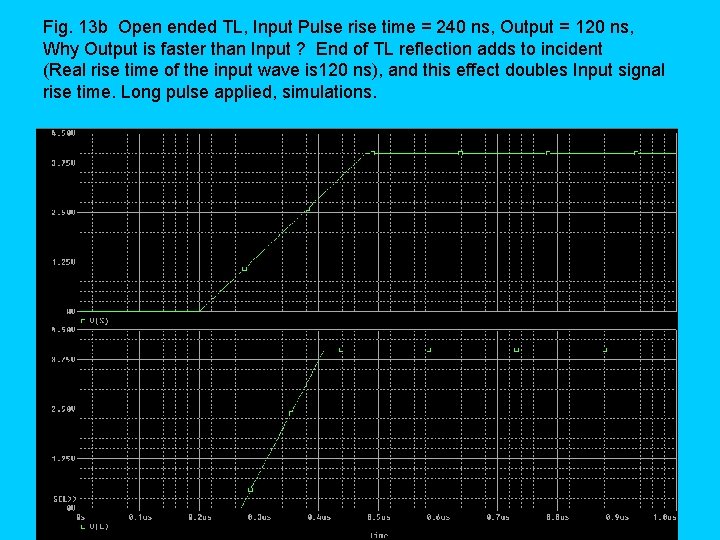
Fig. 13 b Open ended TL, Input Pulse rise time = 240 ns, Output = 120 ns, Why Output is faster than Input ? End of TL reflection adds to incident (Real rise time of the input wave is 120 ns), and this effect doubles Input signal rise time. Long pulse applied, simulations.
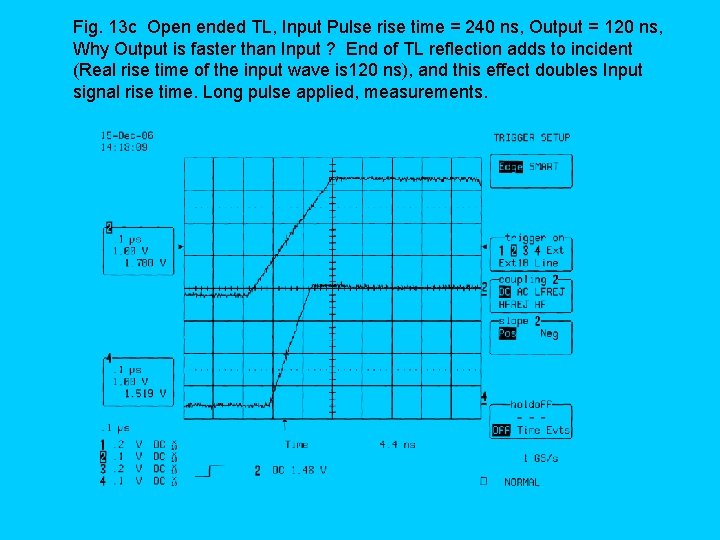
Fig. 13 c Open ended TL, Input Pulse rise time = 240 ns, Output = 120 ns, Why Output is faster than Input ? End of TL reflection adds to incident (Real rise time of the input wave is 120 ns), and this effect doubles Input signal rise time. Long pulse applied, measurements.
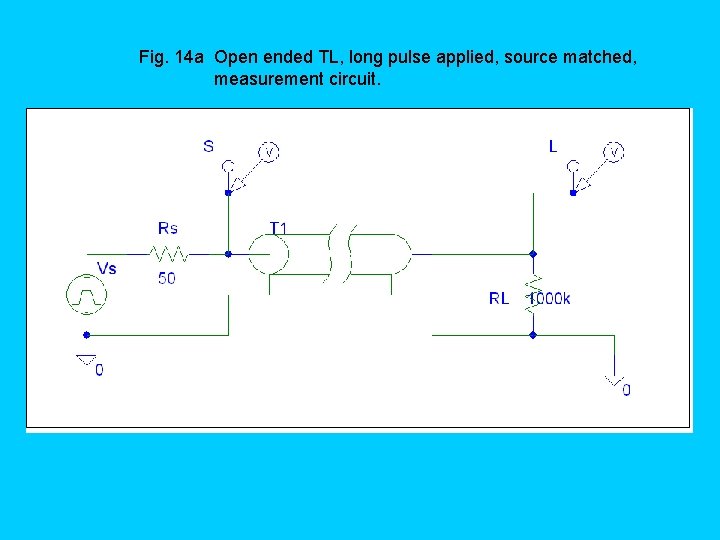
Fig. 14 a Open ended TL, long pulse applied, source matched, measurement circuit.
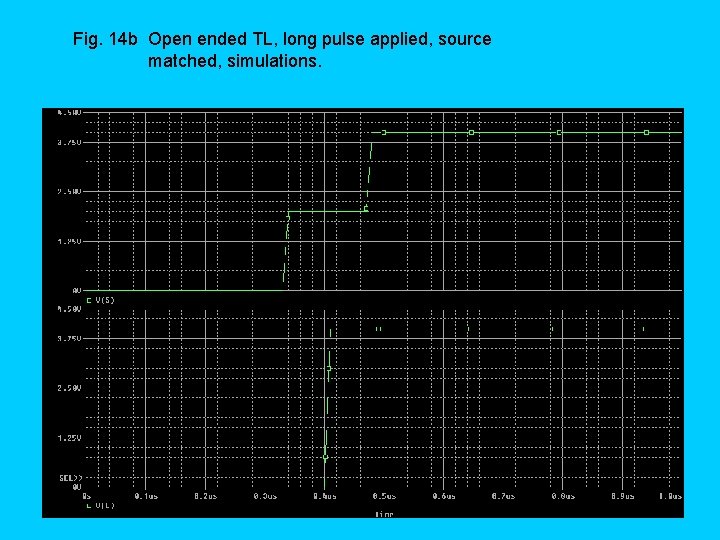
Fig. 14 b Open ended TL, long pulse applied, source matched, simulations.
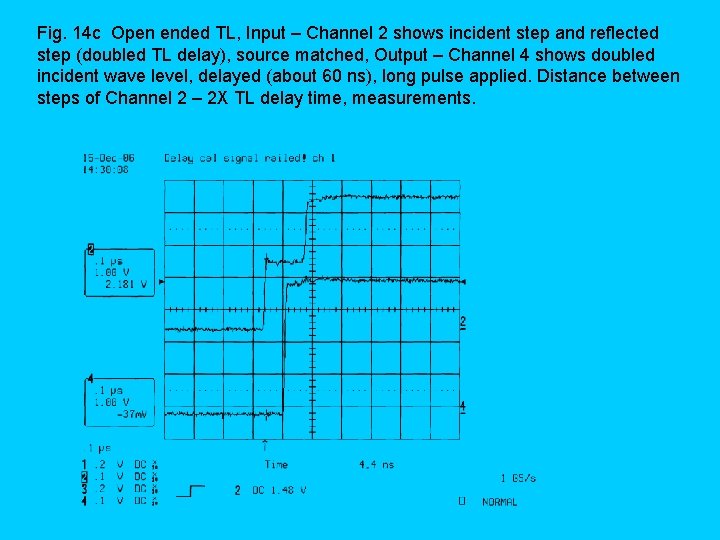
Fig. 14 c Open ended TL, Input – Channel 2 shows incident step and reflected step (doubled TL delay), source matched, Output – Channel 4 shows doubled incident wave level, delayed (about 60 ns), long pulse applied. Distance between steps of Channel 2 – 2 X TL delay time, measurements.
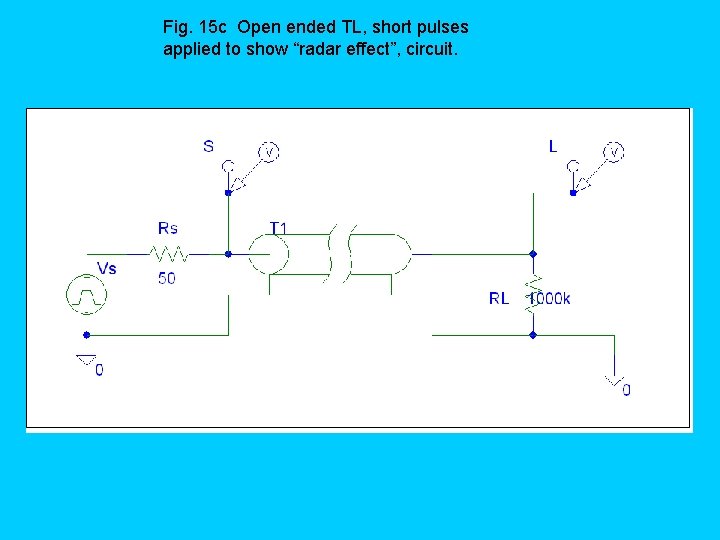
Fig. 15 c Open ended TL, short pulses applied to show “radar effect”, circuit.
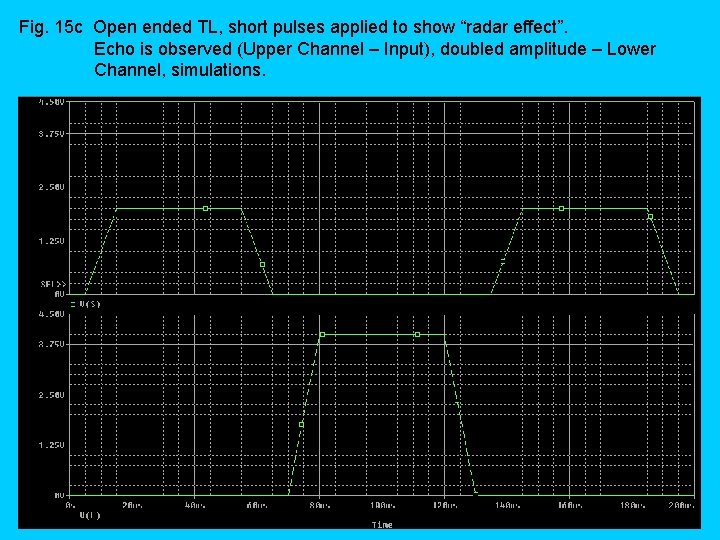
Fig. 15 c Open ended TL, short pulses applied to show “radar effect”. Echo is observed (Upper Channel – Input), doubled amplitude – Lower Channel, simulations.
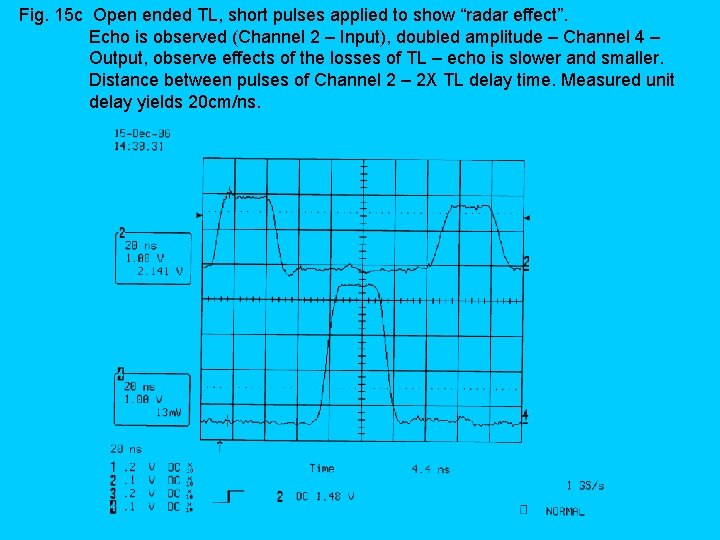
Fig. 15 c Open ended TL, short pulses applied to show “radar effect”. Echo is observed (Channel 2 – Input), doubled amplitude – Channel 4 – Output, observe effects of the losses of TL – echo is slower and smaller. Distance between pulses of Channel 2 – 2 X TL delay time. Measured unit delay yields 20 cm/ns.
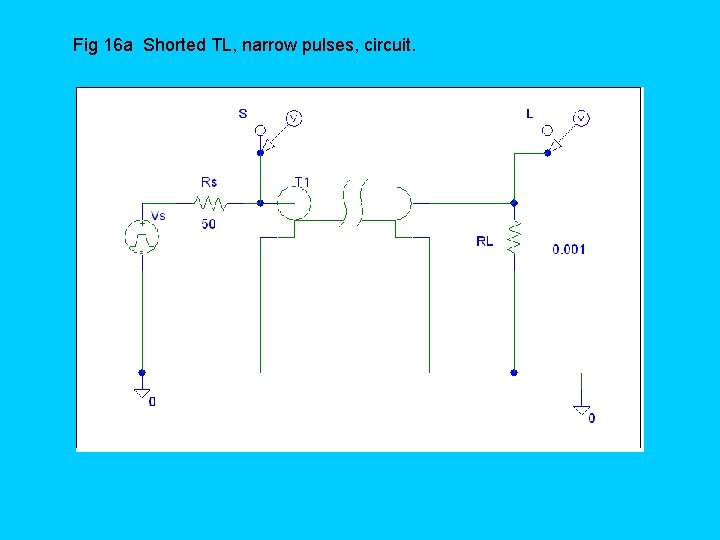
Fig 16 a Shorted TL, narrow pulses, circuit.
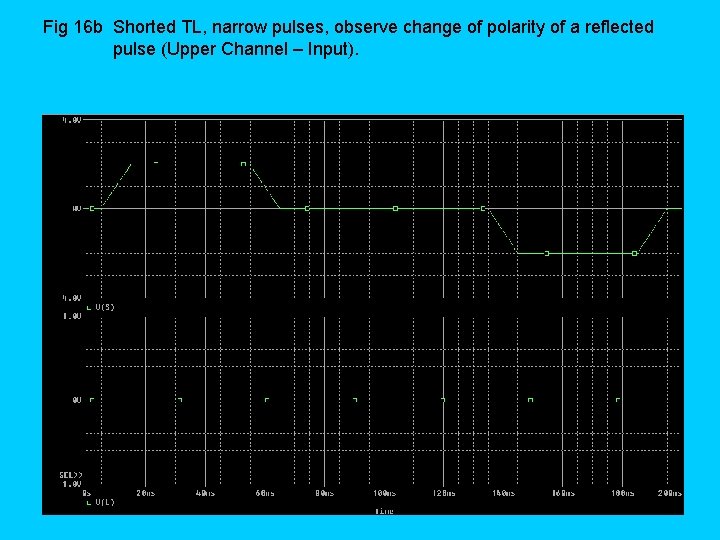
Fig 16 b Shorted TL, narrow pulses, observe change of polarity of a reflected pulse (Upper Channel – Input).
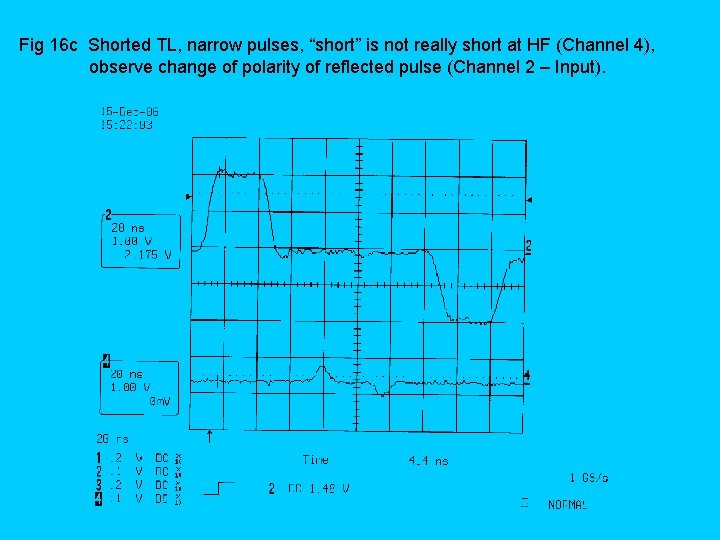
Fig 16 c Shorted TL, narrow pulses, “short” is not really short at HF (Channel 4), observe change of polarity of reflected pulse (Channel 2 – Input).
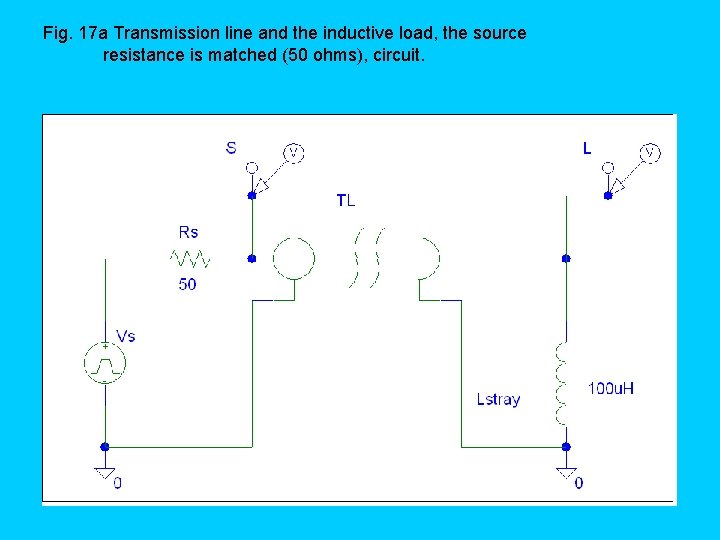
Fig. 17 a Transmission line and the inductive load, the source resistance is matched (50 ohms), circuit.
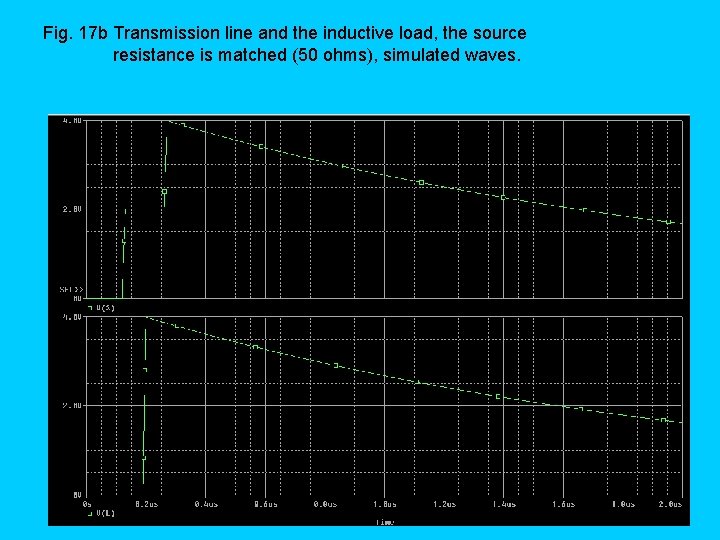
Fig. 17 b Transmission line and the inductive load, the source resistance is matched (50 ohms), simulated waves.
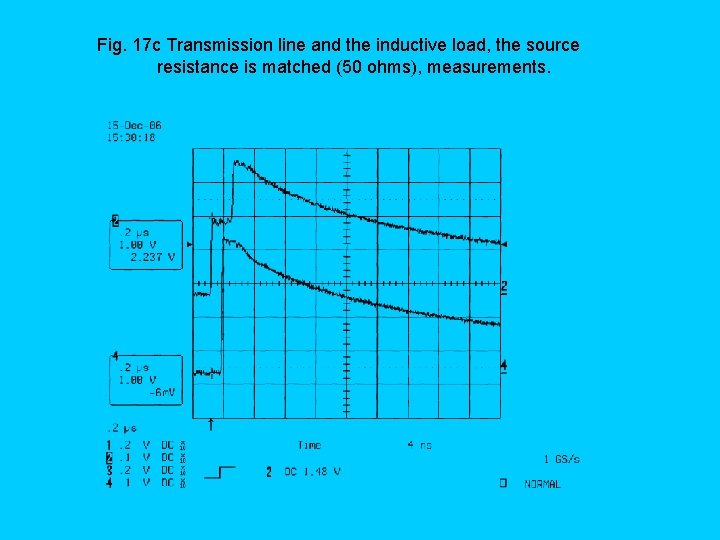
Fig. 17 c Transmission line and the inductive load, the source resistance is matched (50 ohms), measurements.
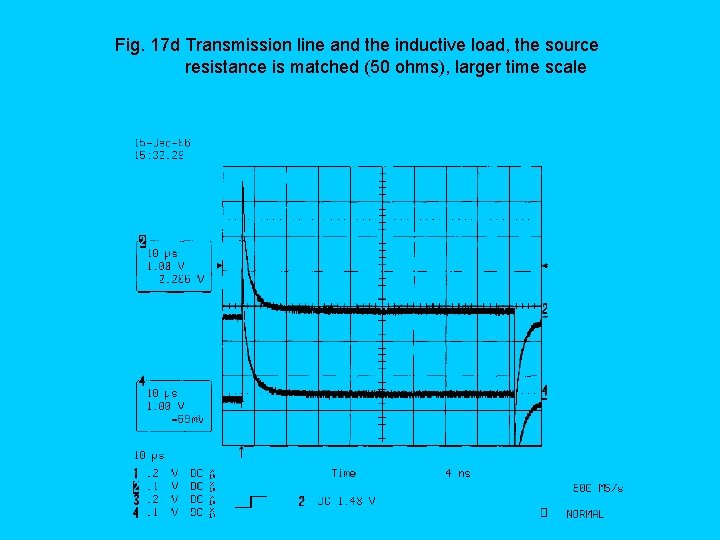
Fig. 17 d Transmission line and the inductive load, the source resistance is matched (50 ohms), larger time scale
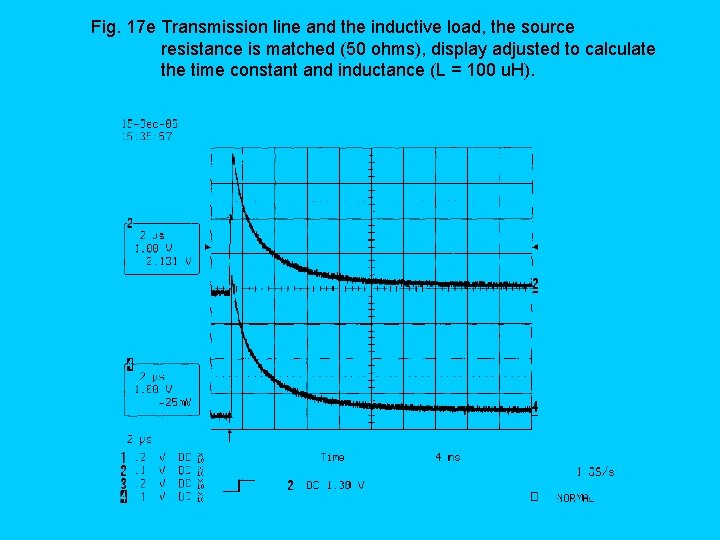
Fig. 17 e Transmission line and the inductive load, the source resistance is matched (50 ohms), display adjusted to calculate the time constant and inductance (L = 100 u. H).
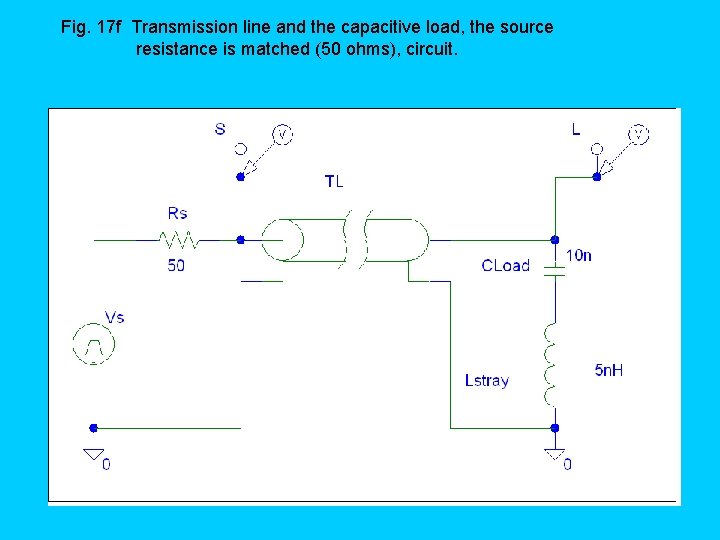
Fig. 17 f Transmission line and the capacitive load, the source resistance is matched (50 ohms), circuit.
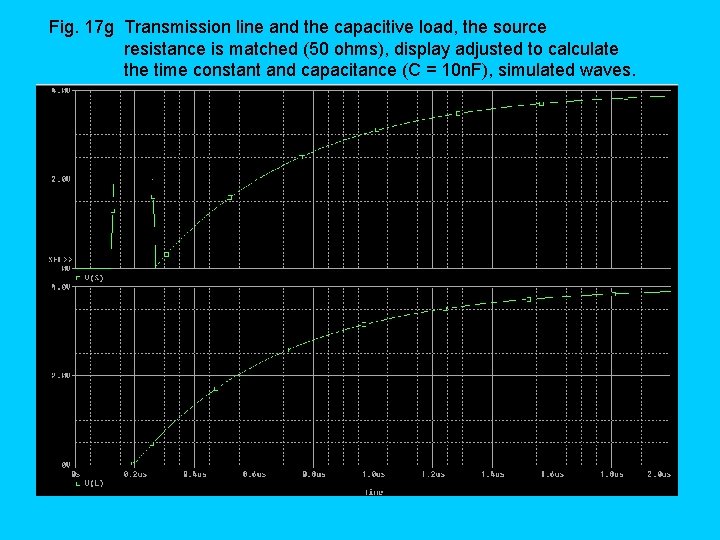
Fig. 17 g Transmission line and the capacitive load, the source resistance is matched (50 ohms), display adjusted to calculate the time constant and capacitance (C = 10 n. F), simulated waves.
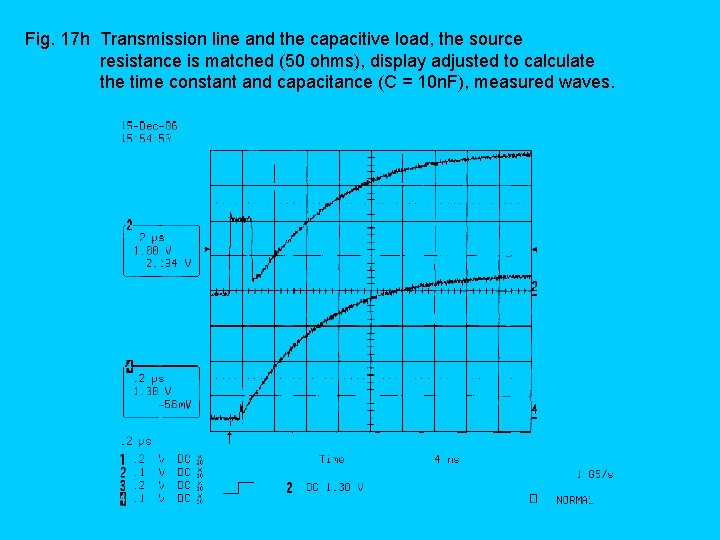
Fig. 17 h Transmission line and the capacitive load, the source resistance is matched (50 ohms), display adjusted to calculate the time constant and capacitance (C = 10 n. F), measured waves.
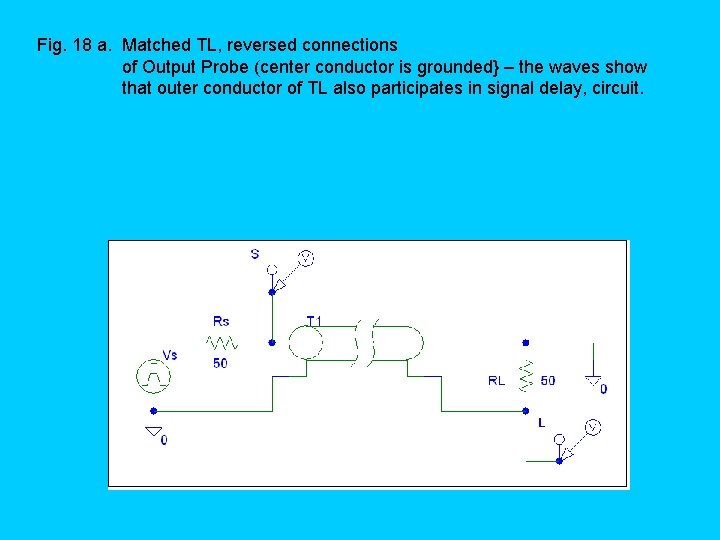
Fig. 18 a. Matched TL, reversed connections of Output Probe (center conductor is grounded} – the waves show that outer conductor of TL also participates in signal delay, circuit.
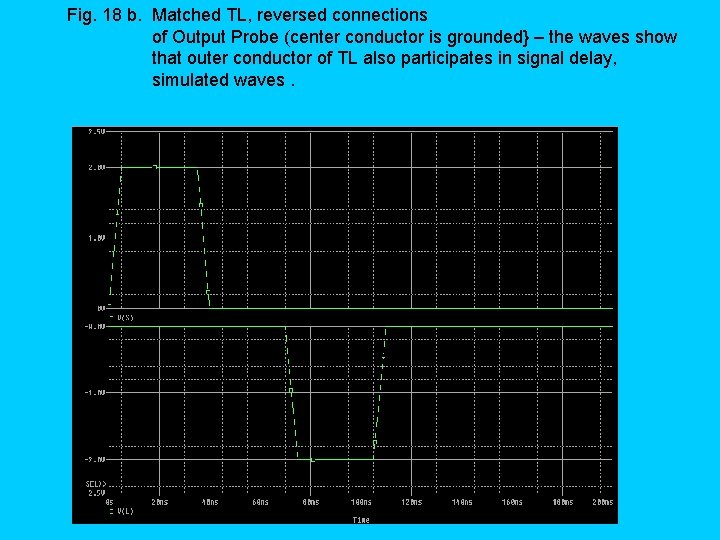
Fig. 18 b. Matched TL, reversed connections of Output Probe (center conductor is grounded} – the waves show that outer conductor of TL also participates in signal delay, simulated waves.
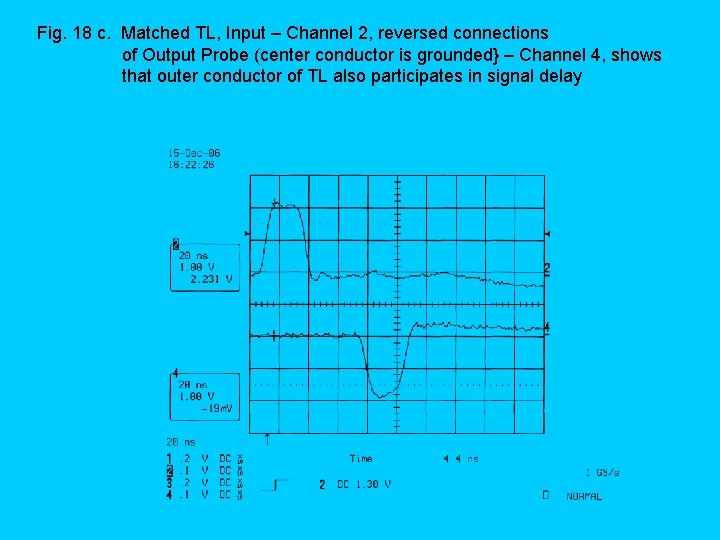
Fig. 18 c. Matched TL, Input – Channel 2, reversed connections of Output Probe (center conductor is grounded} – Channel 4, shows that outer conductor of TL also participates in signal delay
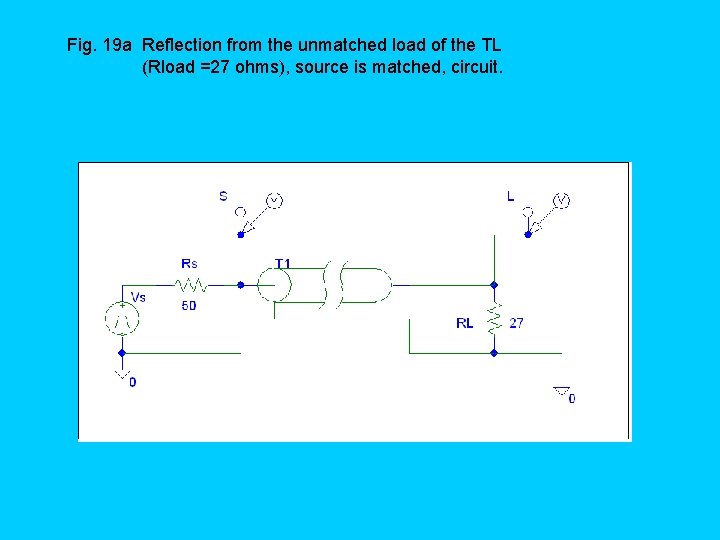
Fig. 19 a Reflection from the unmatched load of the TL (Rload =27 ohms), source is matched, circuit.
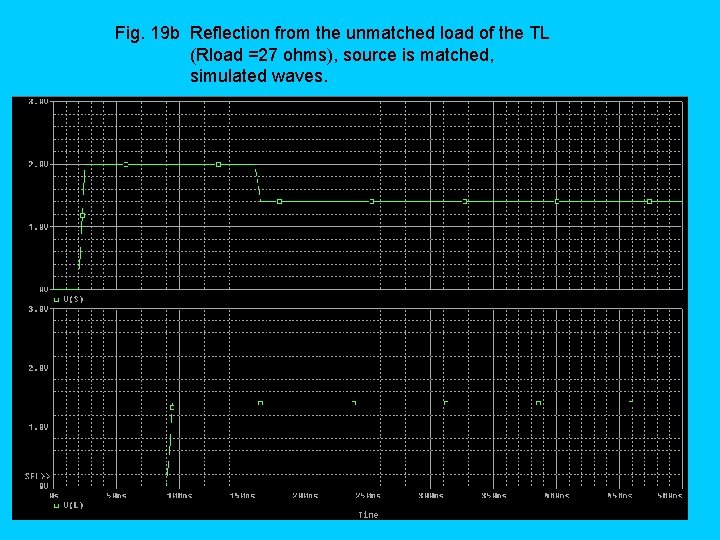
Fig. 19 b Reflection from the unmatched load of the TL (Rload =27 ohms), source is matched, simulated waves.
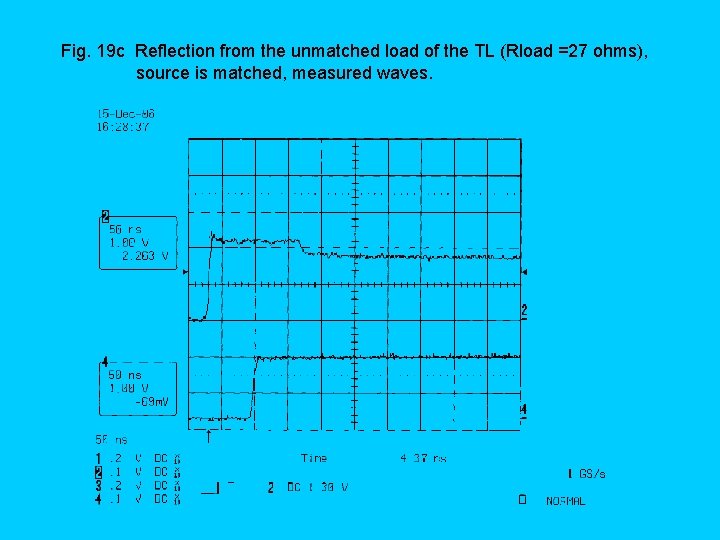
Fig. 19 c Reflection from the unmatched load of the TL (Rload =27 ohms), source is matched, measured waves.
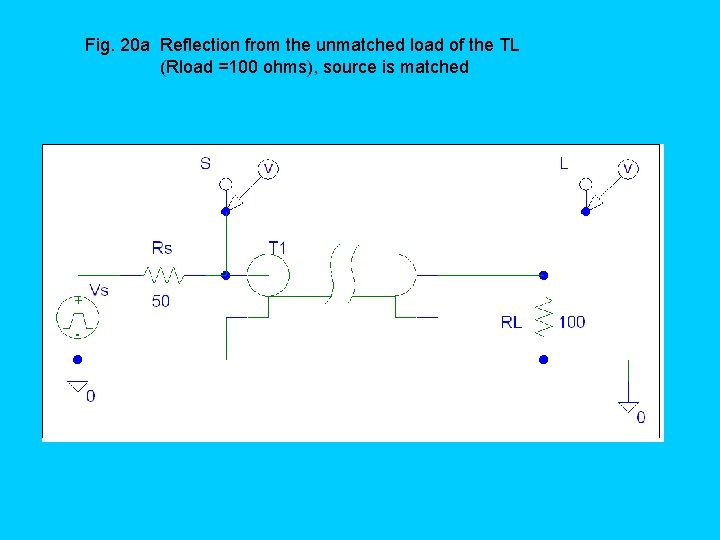
Fig. 20 a Reflection from the unmatched load of the TL (Rload =100 ohms), source is matched
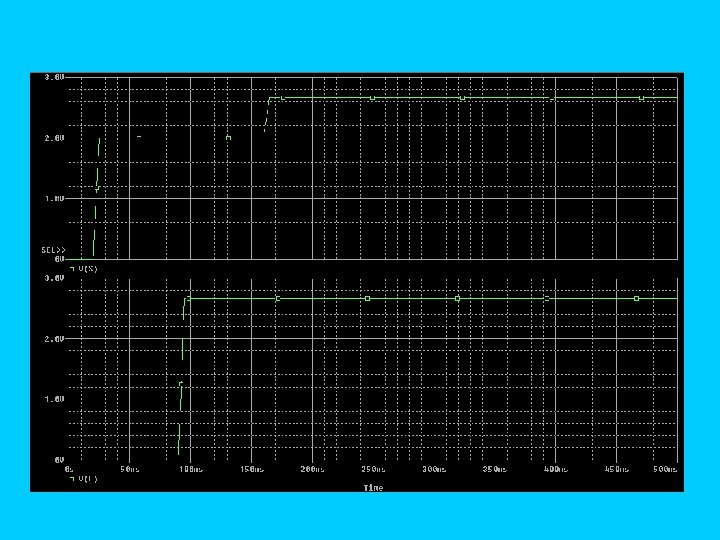
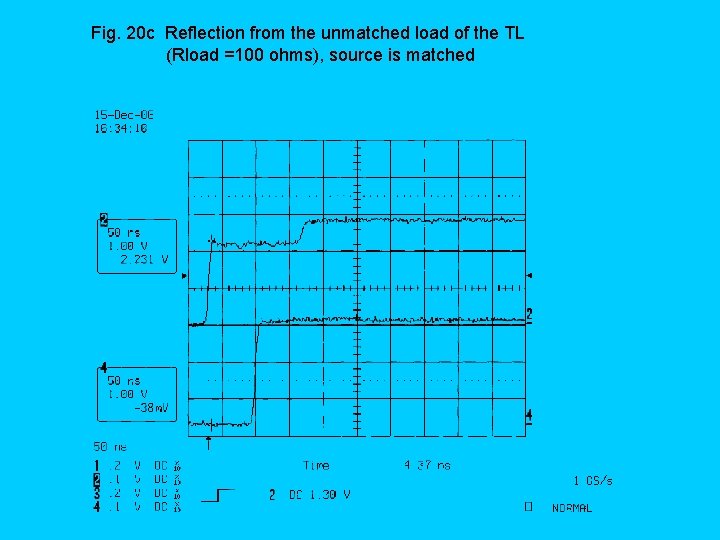
Fig. 20 c Reflection from the unmatched load of the TL (Rload =100 ohms), source is matched
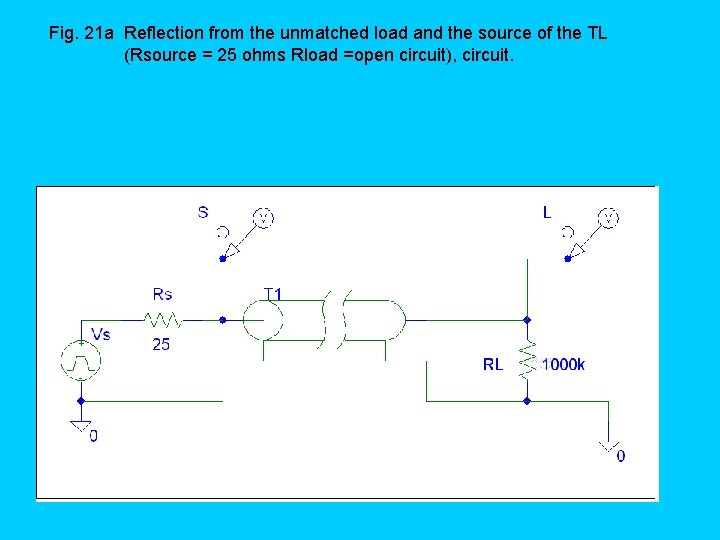
Fig. 21 a Reflection from the unmatched load and the source of the TL (Rsource = 25 ohms Rload =open circuit), circuit.
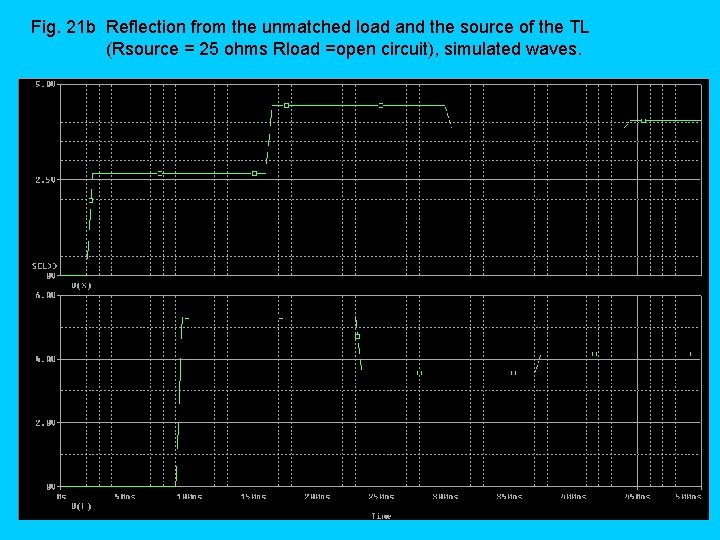
Fig. 21 b Reflection from the unmatched load and the source of the TL (Rsource = 25 ohms Rload =open circuit), simulated waves.
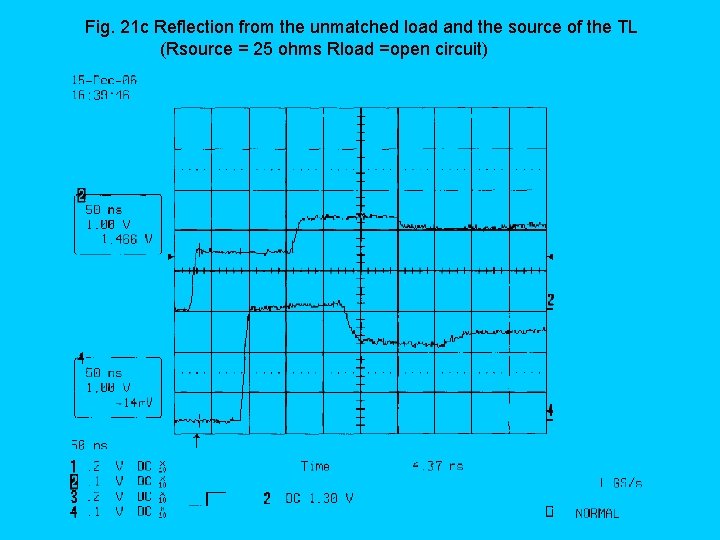
Fig. 21 c Reflection from the unmatched load and the source of the TL (Rsource = 25 ohms Rload =open circuit)
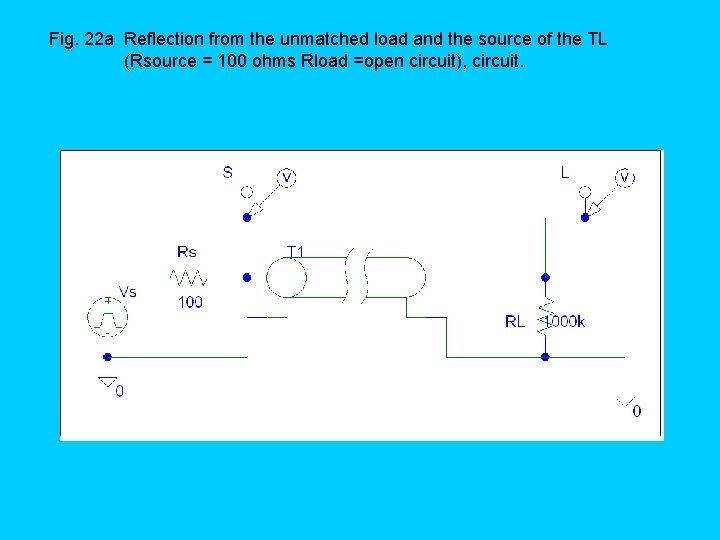
Fig. 22 a Reflection from the unmatched load and the source of the TL (Rsource = 100 ohms Rload =open circuit), circuit.
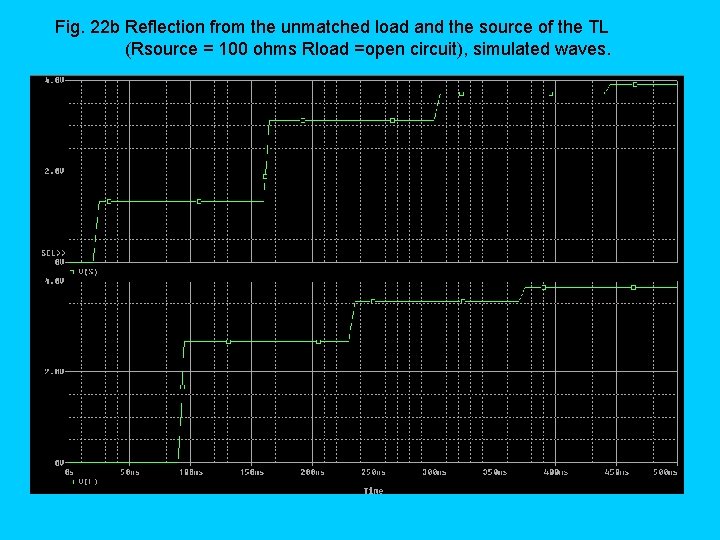
Fig. 22 b Reflection from the unmatched load and the source of the TL (Rsource = 100 ohms Rload =open circuit), simulated waves.
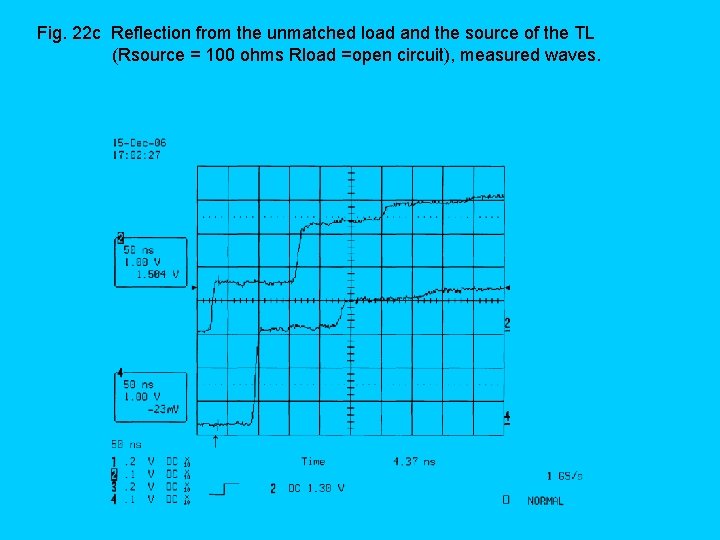
Fig. 22 c Reflection from the unmatched load and the source of the TL (Rsource = 100 ohms Rload =open circuit), measured waves.
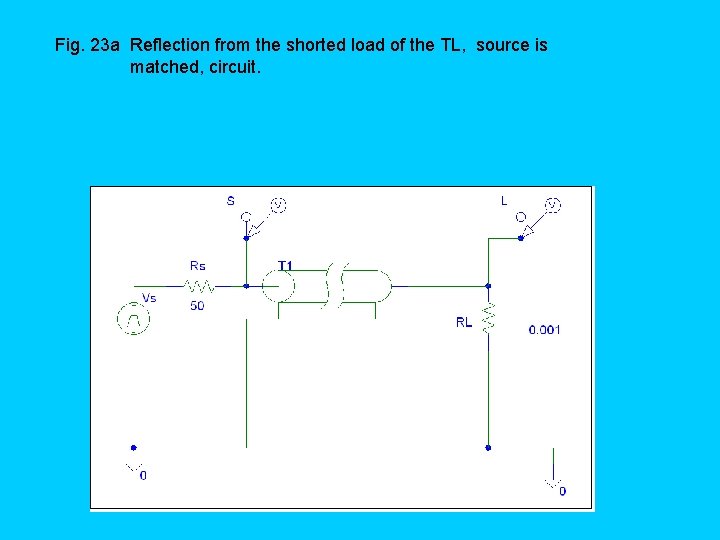
Fig. 23 a Reflection from the shorted load of the TL, source is matched, circuit.
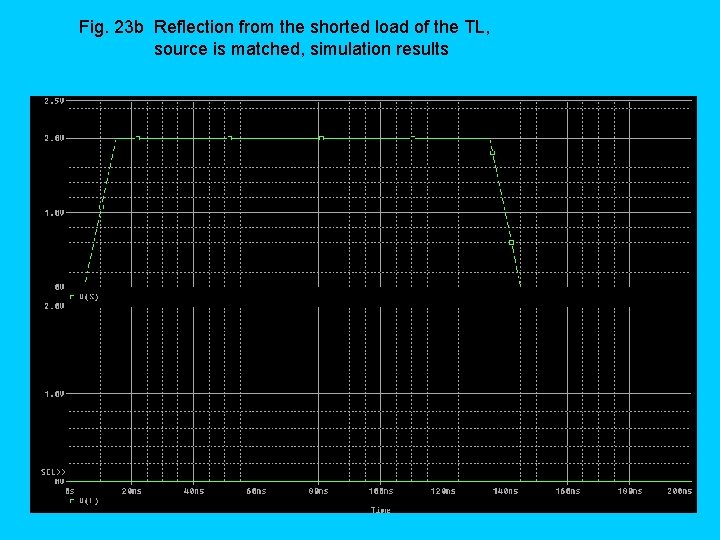
Fig. 23 b Reflection from the shorted load of the TL, source is matched, simulation results
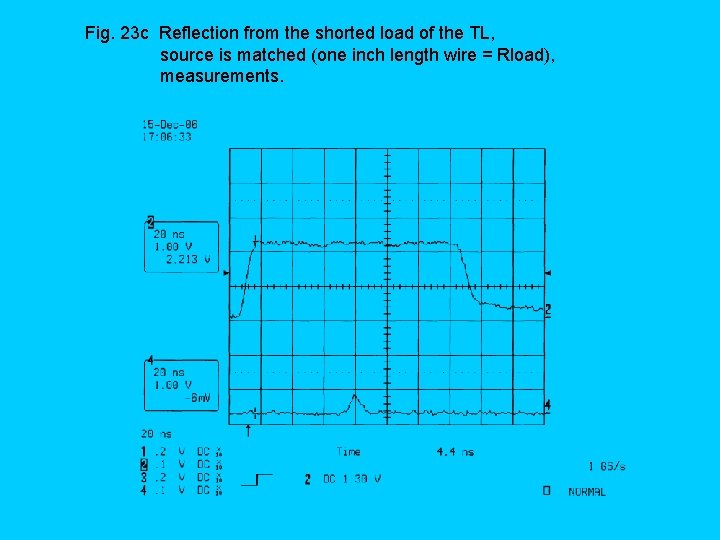
Fig. 23 c Reflection from the shorted load of the TL, source is matched (one inch length wire = Rload), measurements.
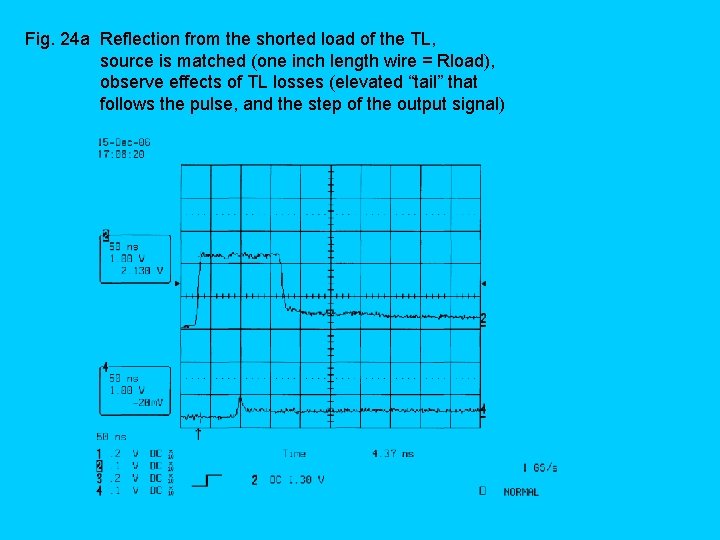
Fig. 24 a Reflection from the shorted load of the TL, source is matched (one inch length wire = Rload), observe effects of TL losses (elevated “tail” that follows the pulse, and the step of the output signal)
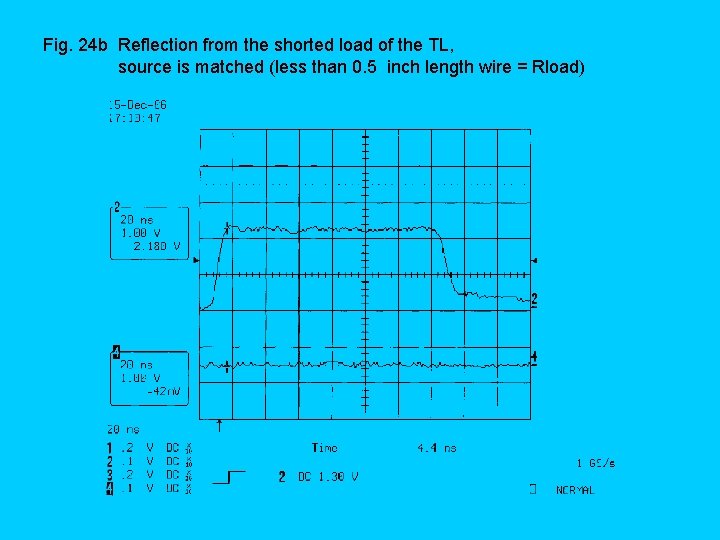
Fig. 24 b Reflection from the shorted load of the TL, source is matched (less than 0. 5 inch length wire = Rload)
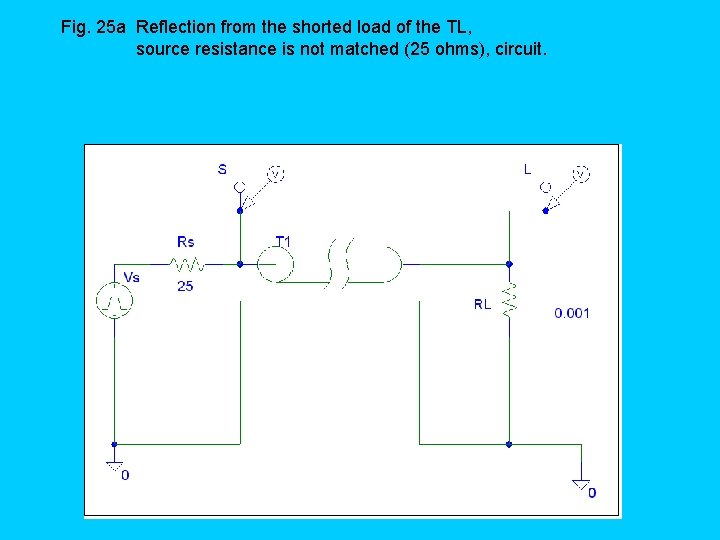
Fig. 25 a Reflection from the shorted load of the TL, source resistance is not matched (25 ohms), circuit.
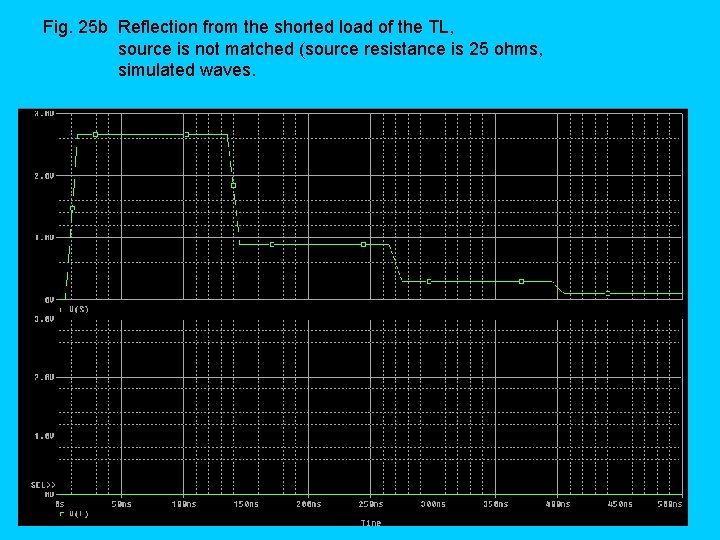
Fig. 25 b Reflection from the shorted load of the TL, source is not matched (source resistance is 25 ohms, simulated waves.
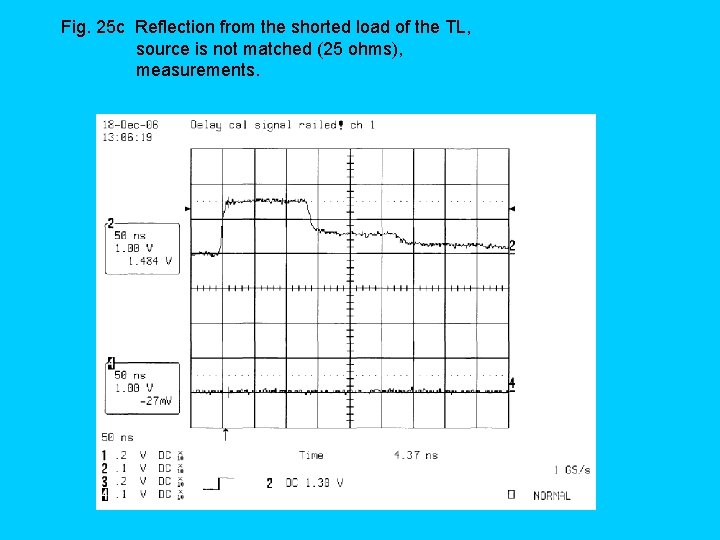
Fig. 25 c Reflection from the shorted load of the TL, source is not matched (25 ohms), measurements.
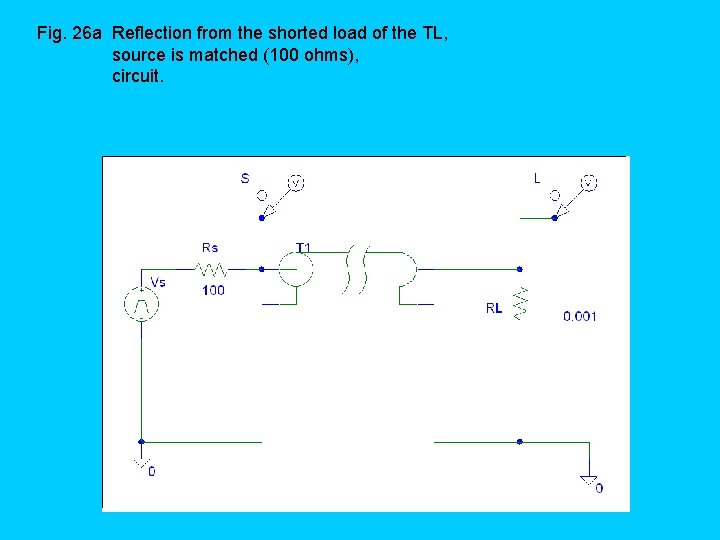
Fig. 26 a Reflection from the shorted load of the TL, source is matched (100 ohms), circuit.
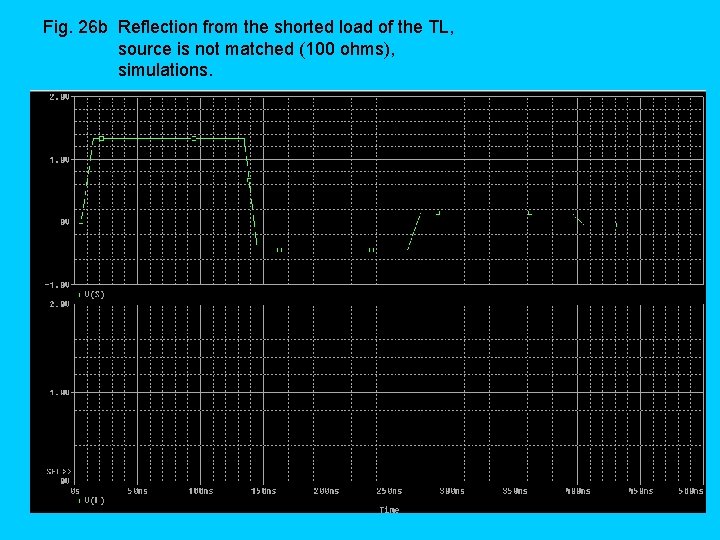
Fig. 26 b Reflection from the shorted load of the TL, source is not matched (100 ohms), simulations.
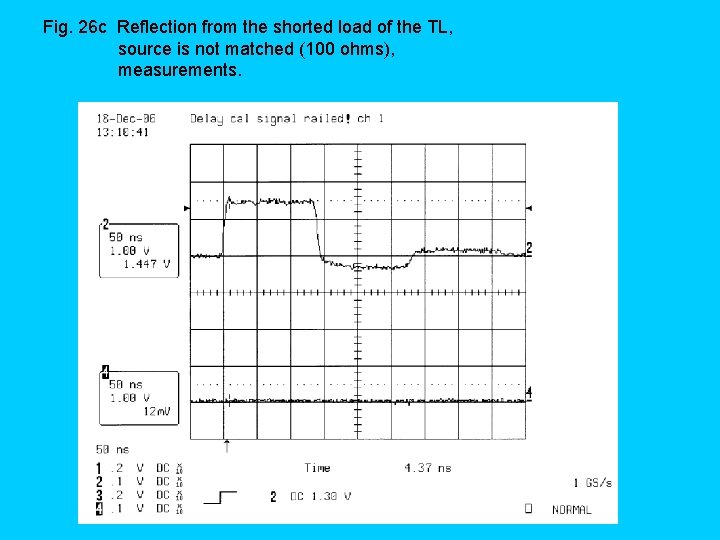
Fig. 26 c Reflection from the shorted load of the TL, source is not matched (100 ohms), measurements.
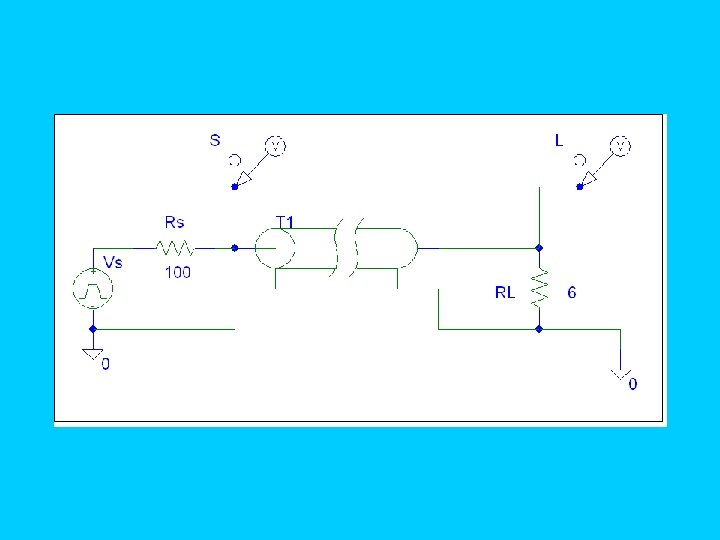
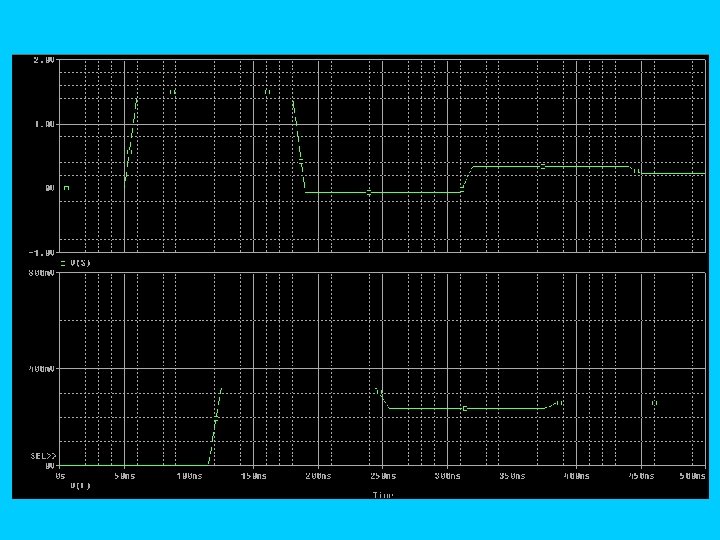
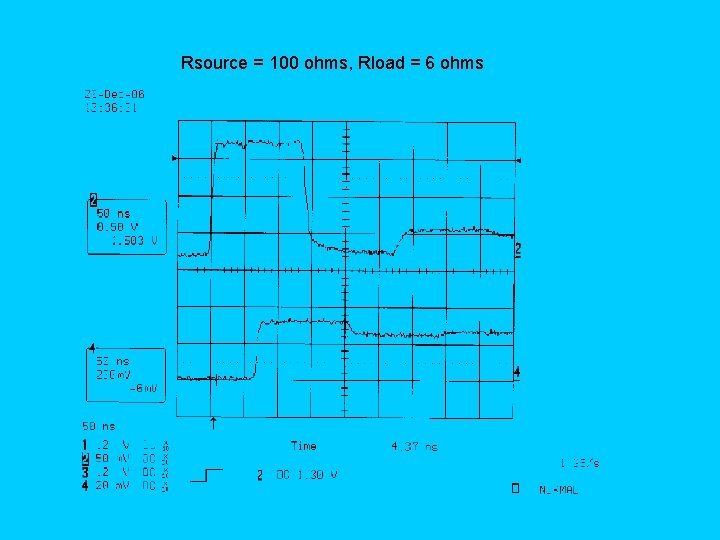
Rsource = 100 ohms, Rload = 6 ohms
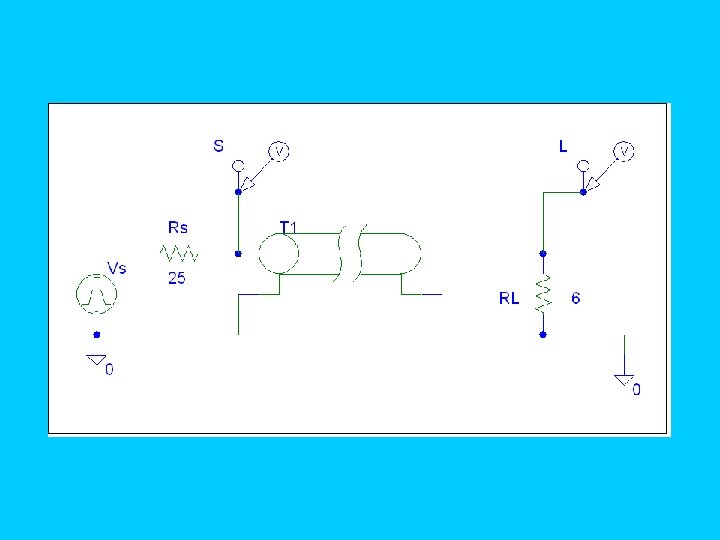
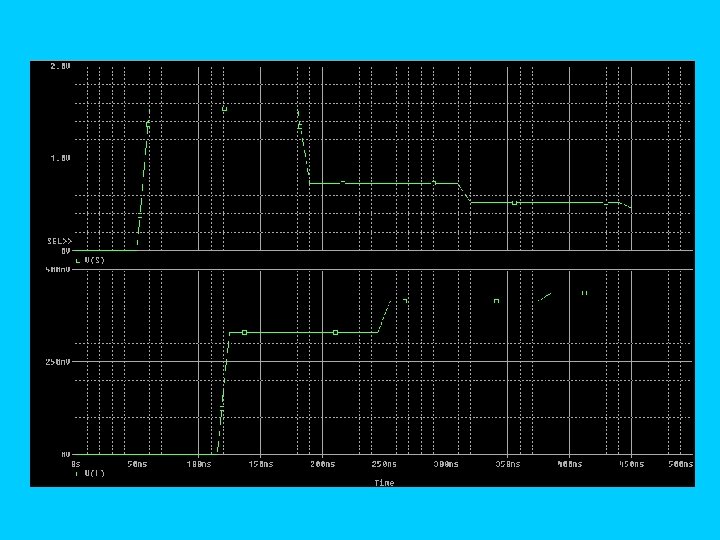
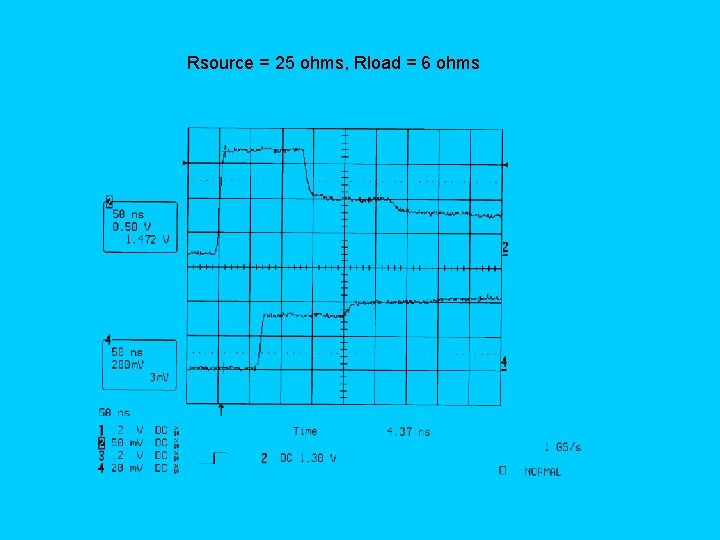
Rsource = 25 ohms, Rload = 6 ohms
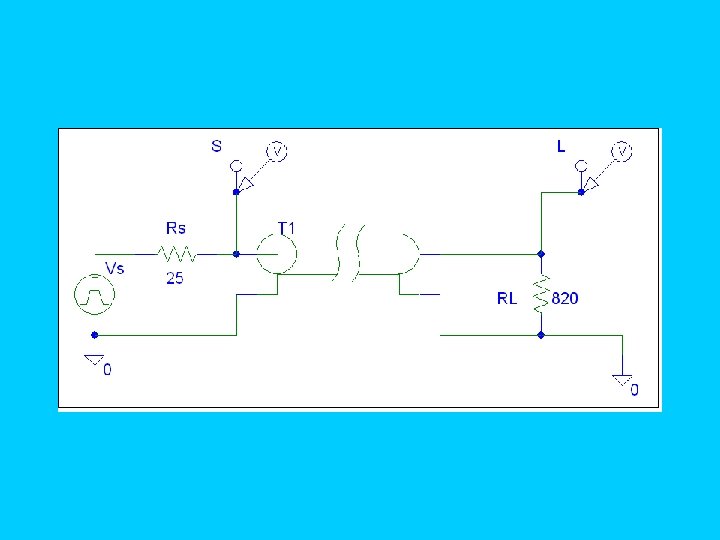
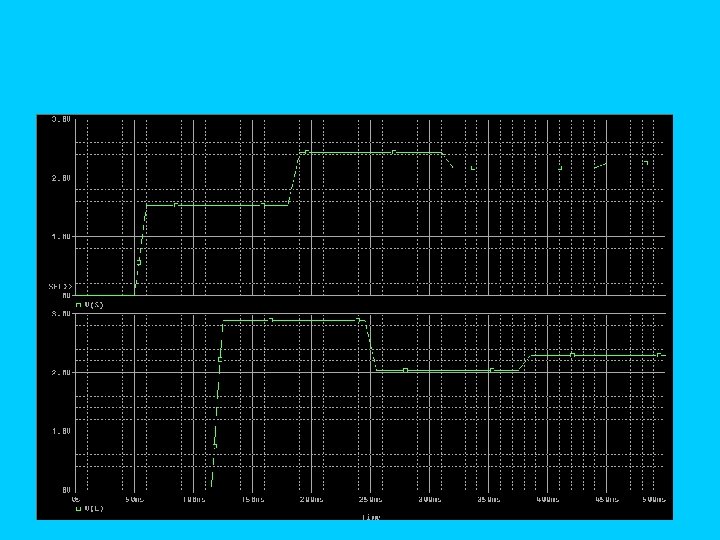
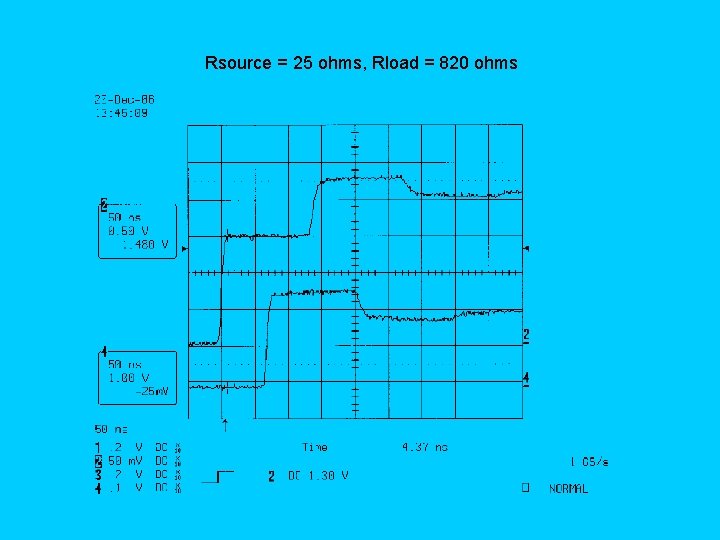
Rsource = 25 ohms, Rload = 820 ohms
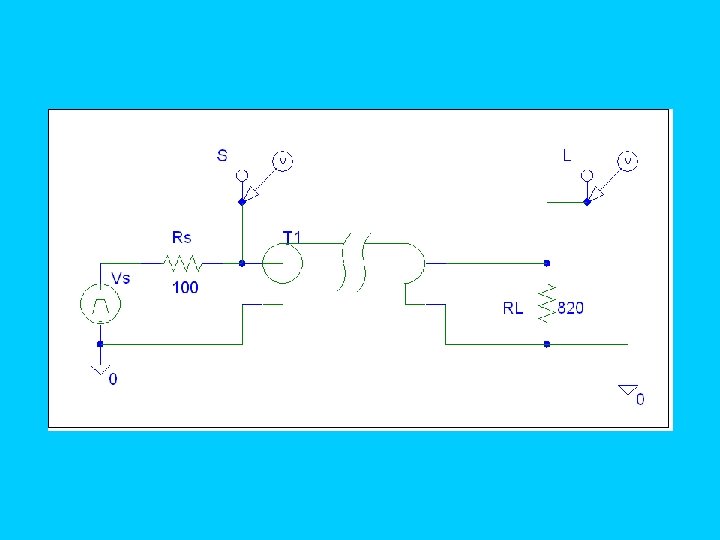
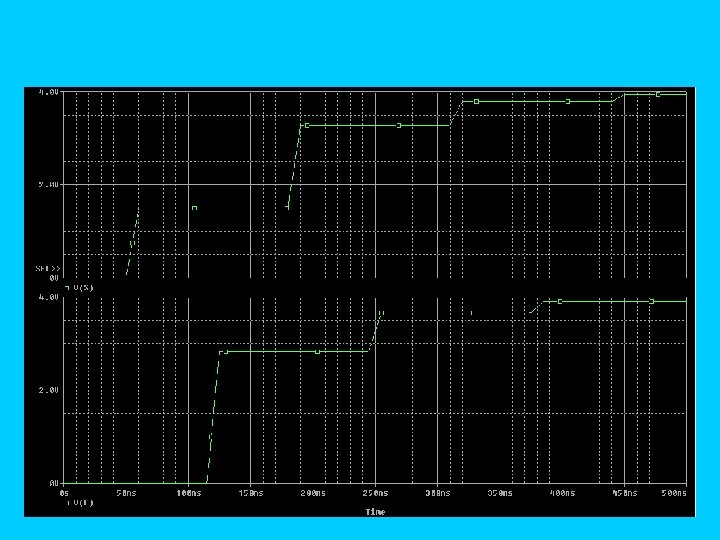
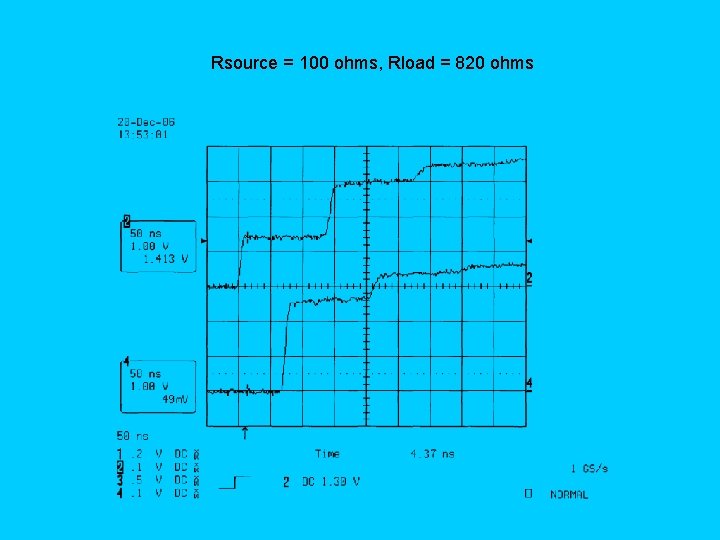
Rsource = 100 ohms, Rload = 820 ohms
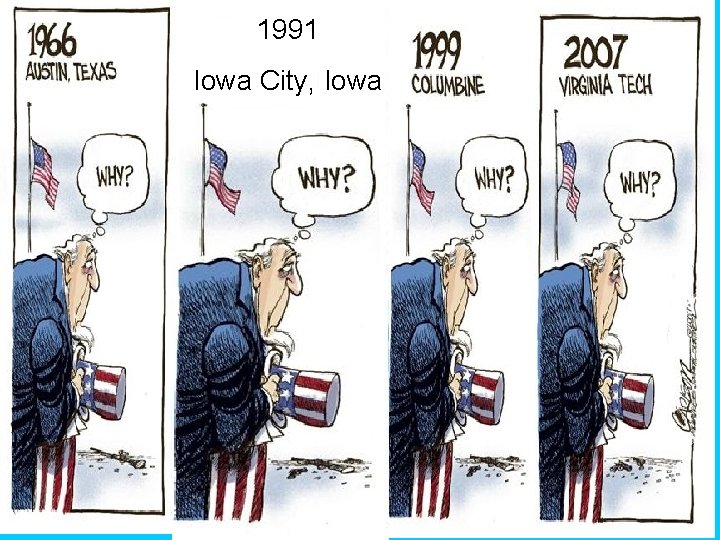
1991 Iowa City, Iowa
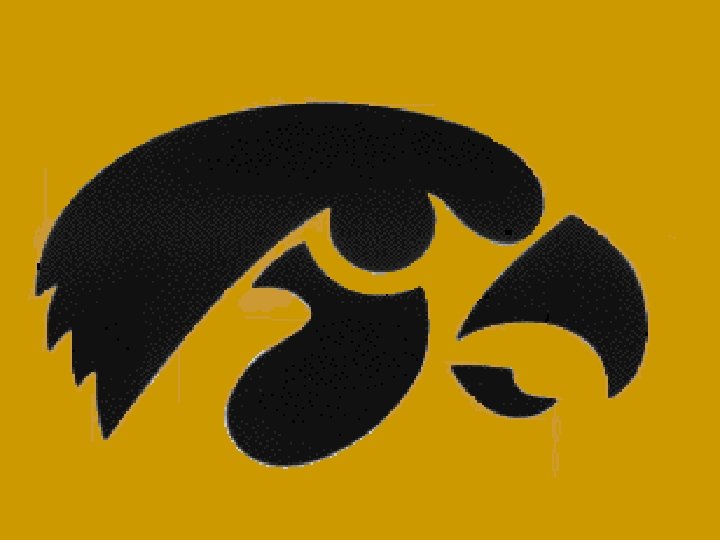
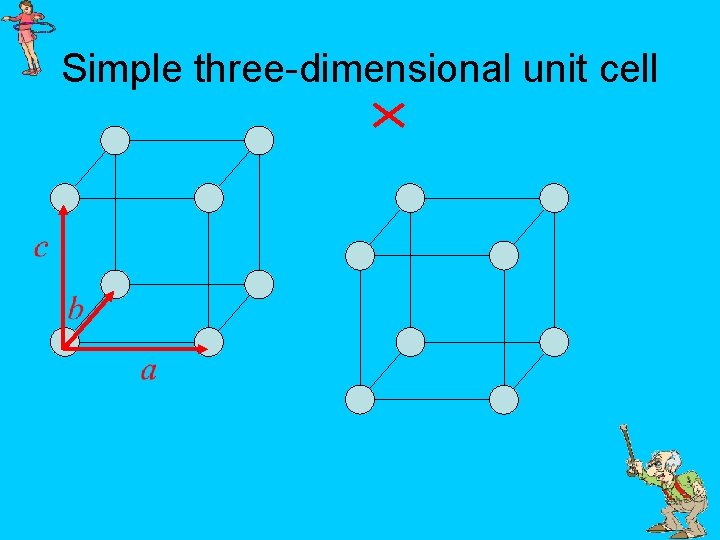
Simple three-dimensional unit cell
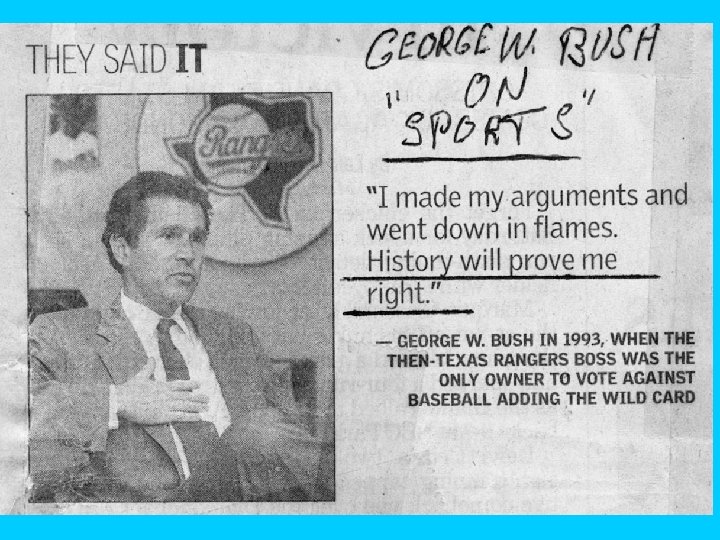
Mos cmos
Cmos inverter analysis
Fabrication steps of cmos inverter
Cmos inverter vtc
Cmos short circuit current
Regenerative property of cmos inverter
Cmos inverter small signal model
Cmos inverter vtc
Layout
In cmos inverters, super-buffers have
Complementary mos
Ganged cmos logic
Cmos soi
For complex gate design in cmos or
Clocked cmos latch
Layout design rules in vlsi
Skewed gates
Distributed rc delay model
Standart cmos setup
2 input nand gate using pass transistor logic
Cmos rfic
Anigay cmos
Intel cmos
Ccd dsk
Cmos op amp
Soi vs bulk cmos
Pmos nand
Rs flip flop cmos
Standard cmos features
Wpe effect in layout
Cmos cross section
Dynamic nand gate
Poly resistor layout
N+ polysilicon
Cmos
Cmos technology
Euler path cmos
Gsense400bsi
Device modeling for analog and rf cmos circuit design
Cmos certification
Latchup in cmos
Cmos process flow
Cmos
Cmos
Razavi cmos analog circuit design
Cmos vlsi design lecture notes
Nwell
Characteristics of logic family
Cmos op amp
Ttl cmos ecl comparison table
Cmos lc oscillator
Cmos vs ttl
Cmos
Y=a+bc using cmos
Cmos backplane
Ic mosfet amplifiers
Eder chavez
Fanin and fanout in cmos
Skewed gates
Cmos technology
Cvsl in vlsi
Layout of analog cmos ic maloberti
Synchronous dynamic circuit techniques
Rtl inverter
Differential amplifier example problems
Gates using cmos
Cmos amplifier
Diode layout in cmos
Otp fuse
Cmos vtc
Mos sensor panasonic
Mos vt
Mos transistor theory
Moscap
Osmo vertigazon
Mos que
Mos c-v
Mos
Mos varactor
Gfs lamp meteogram
Nmos iv curve
Examples of mos and sds
Mos miron prisacarul
Comenius
Caracterizarea personajului principal enigma otiliei
Frugalitas mos maiorum
Mos text bulletins
Mos monza
Ideal mos capacitor
Usmc ecfc
Mosfet differential amplifier
Jana gajusová
Mos varactor
Moral berasal dari bahasa latin “mos” yang berarti
Hlg-mos
C-v characteristics of mos capacitor
Ci mos
Mos video quality
Mos transistor
Mos
Mos moris
Sai infotech