UNIT 5 THERMODYNAMICS THERMOCHEMISTRY Ms Di Orio Rm
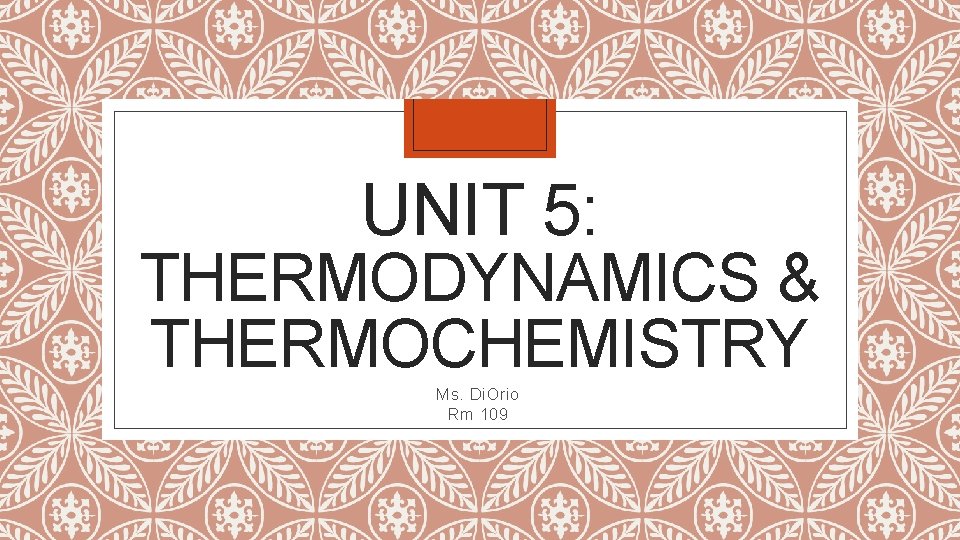
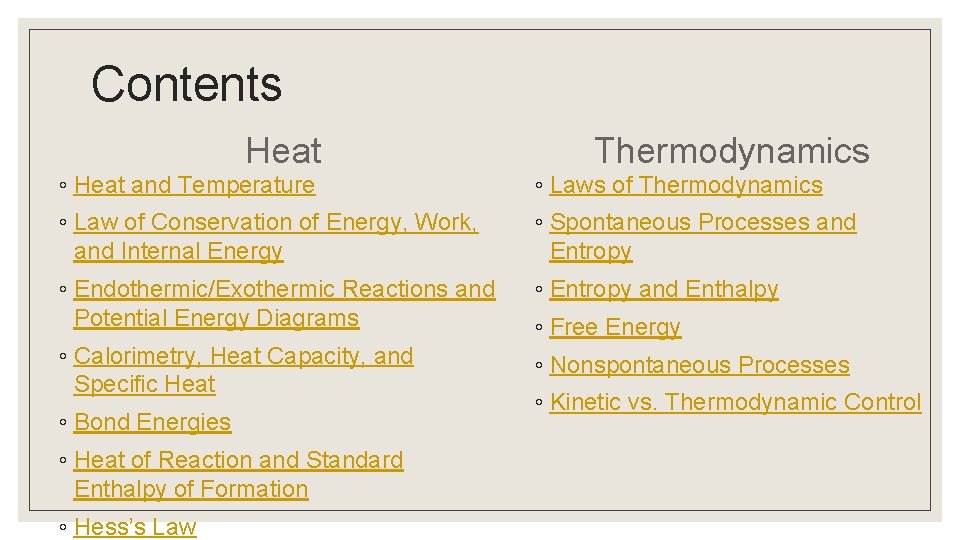
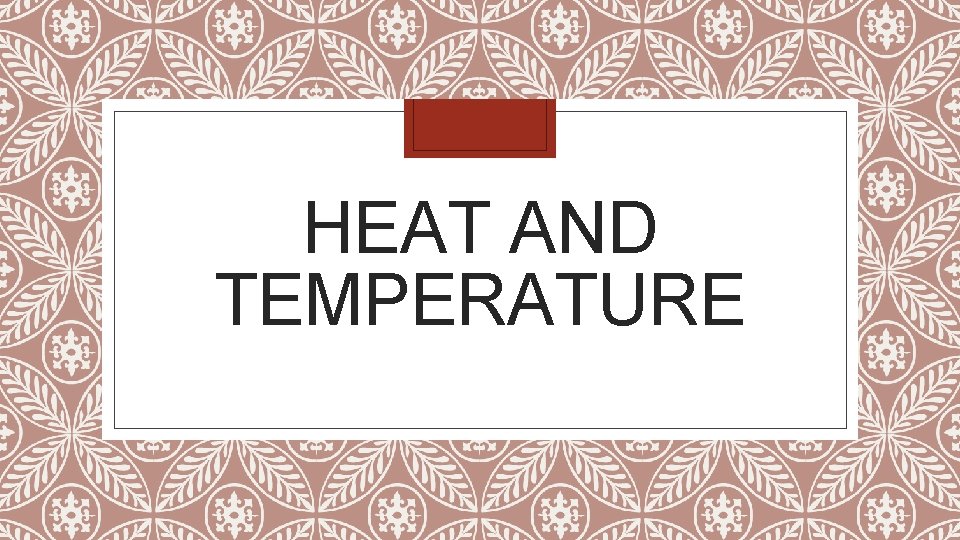
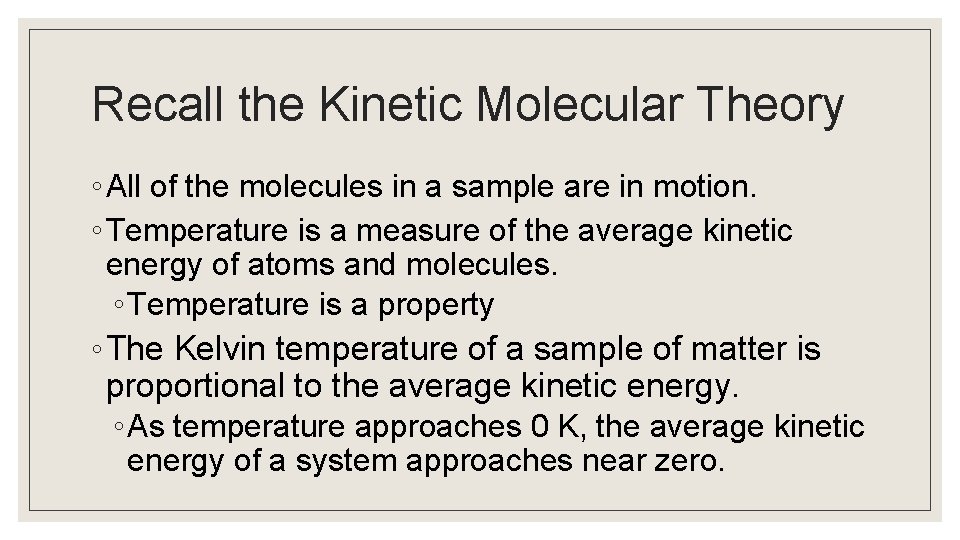
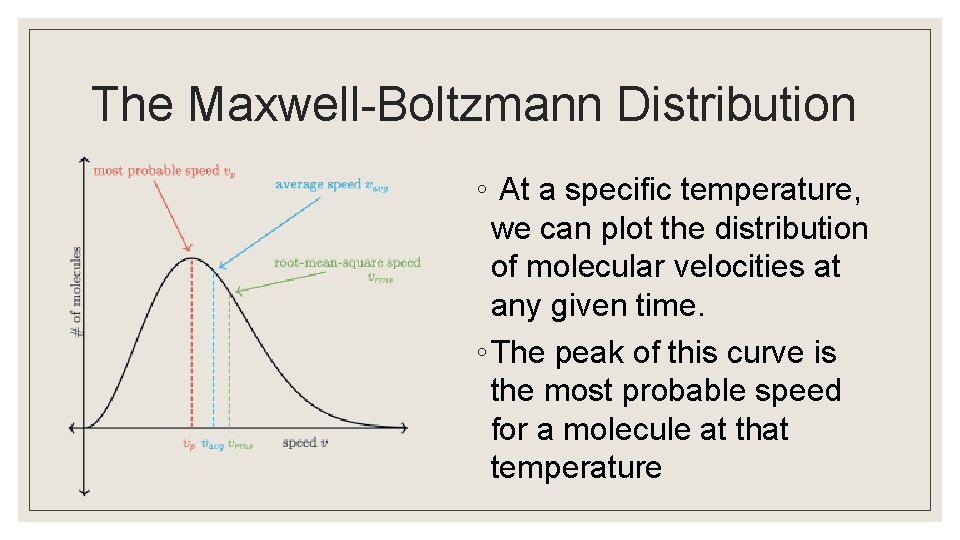
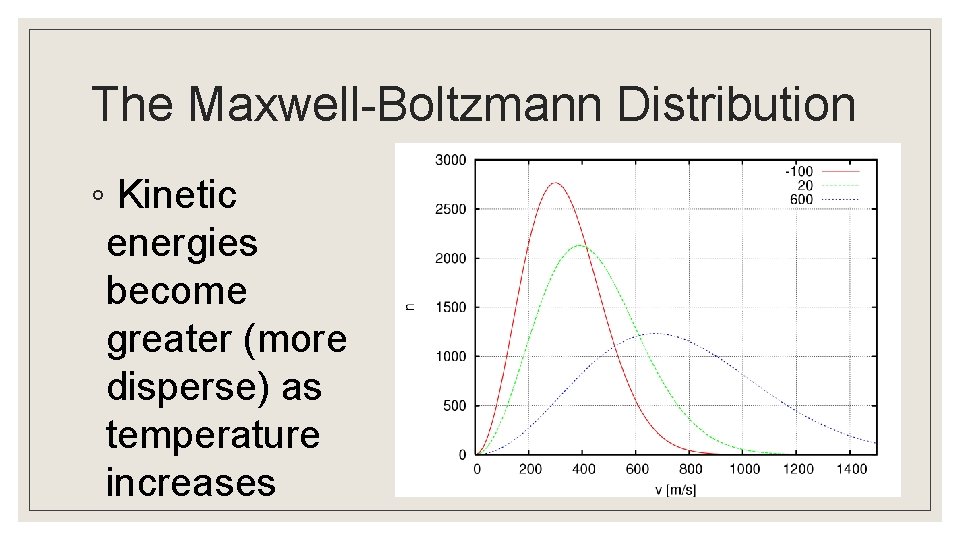
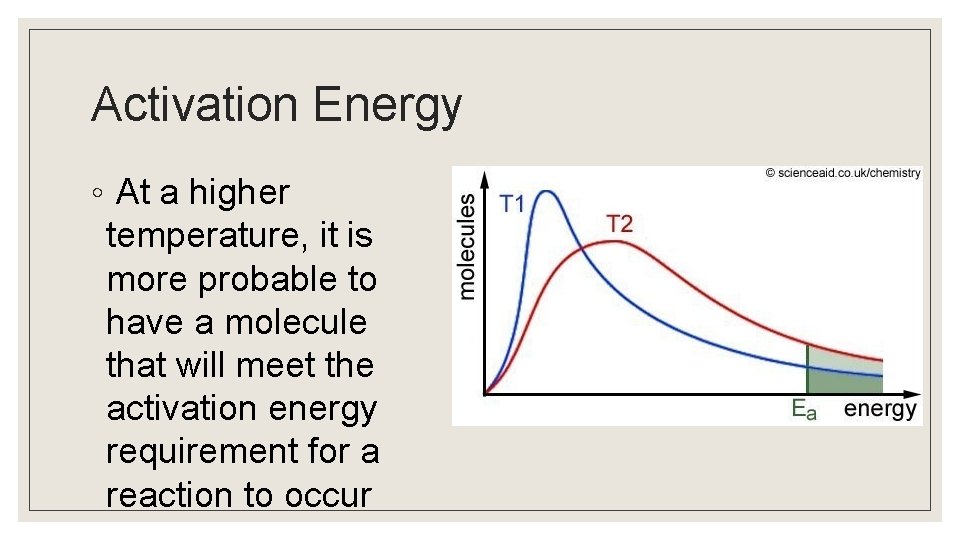
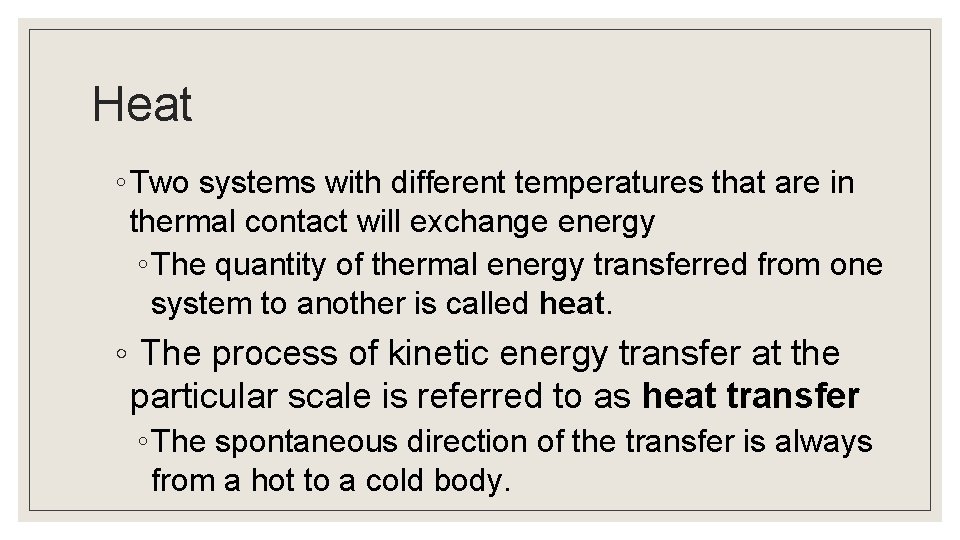
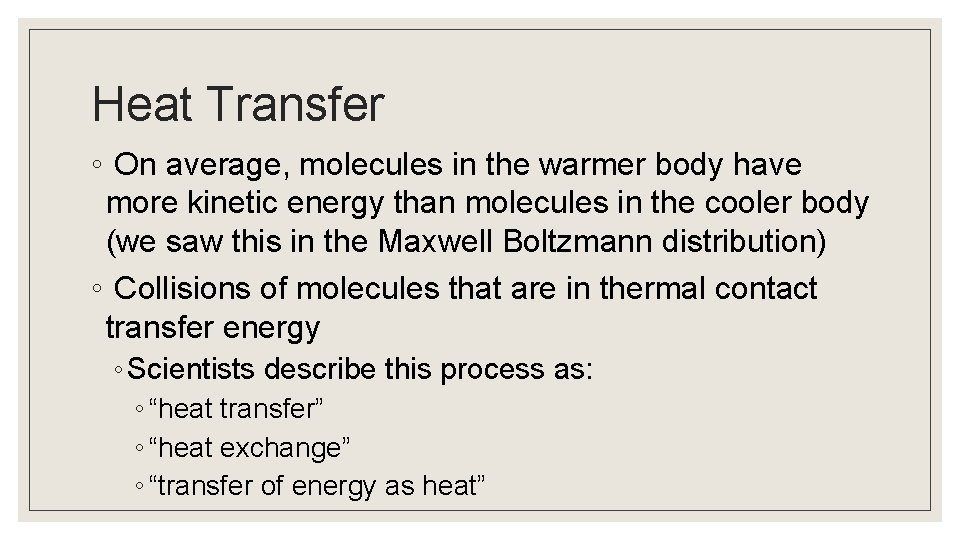
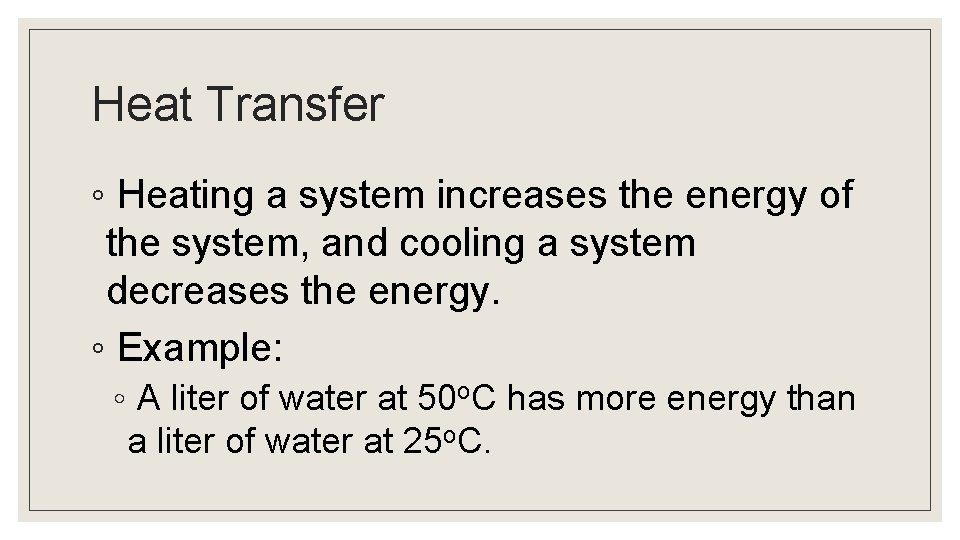
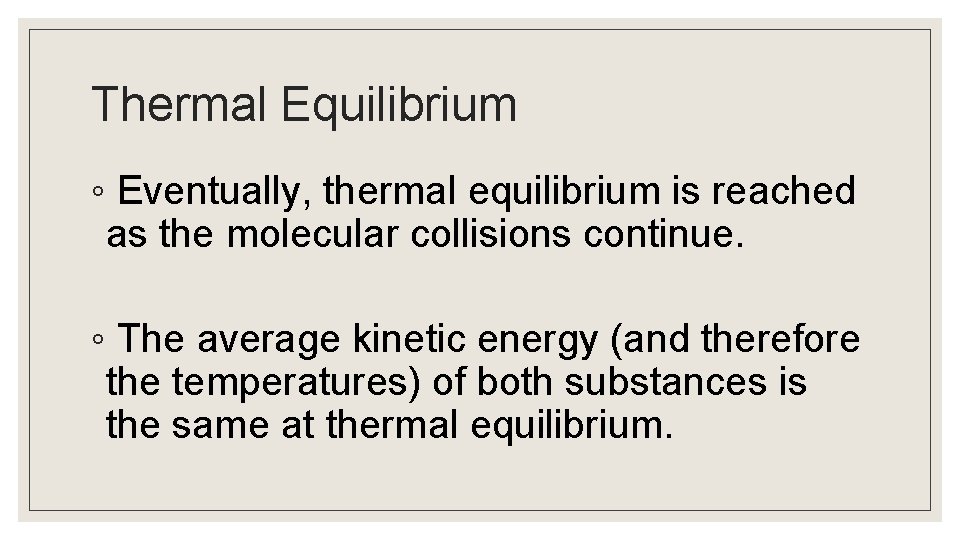
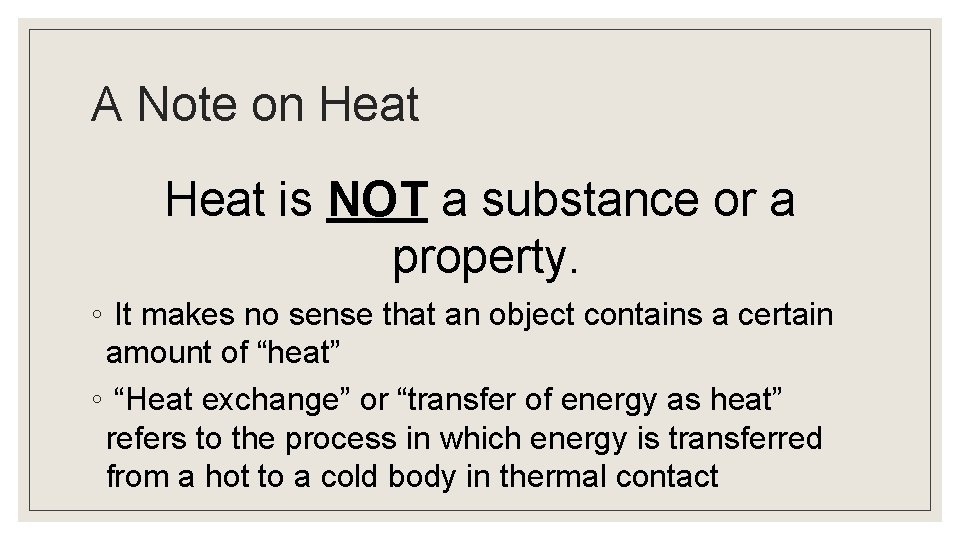
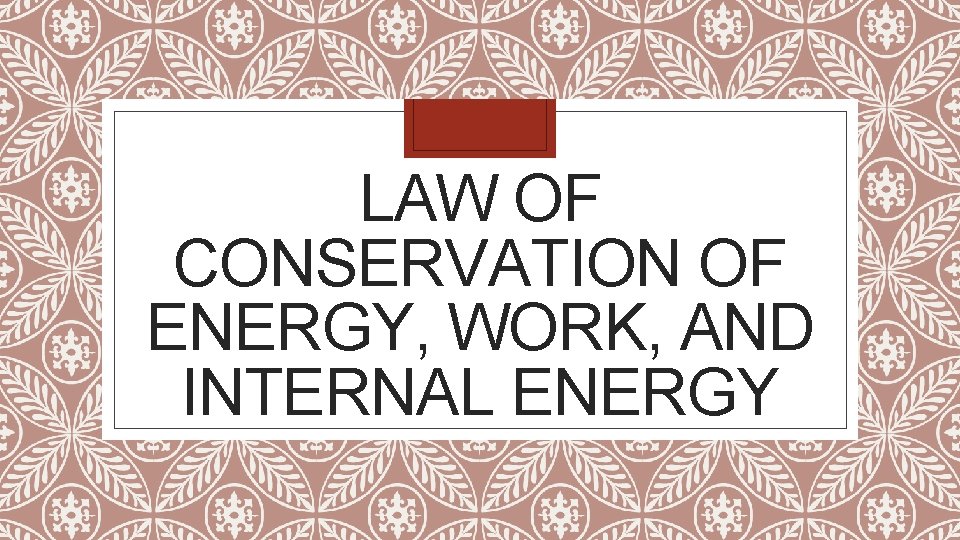
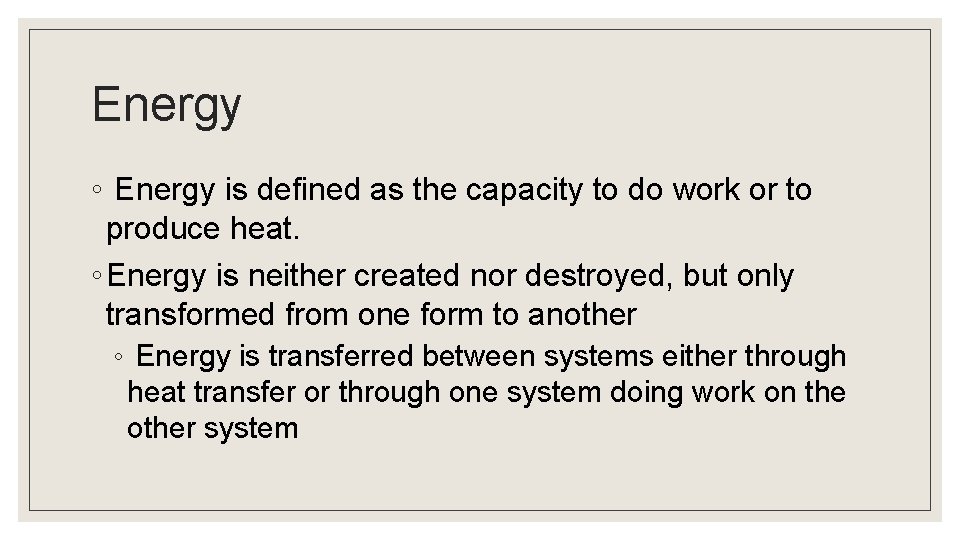
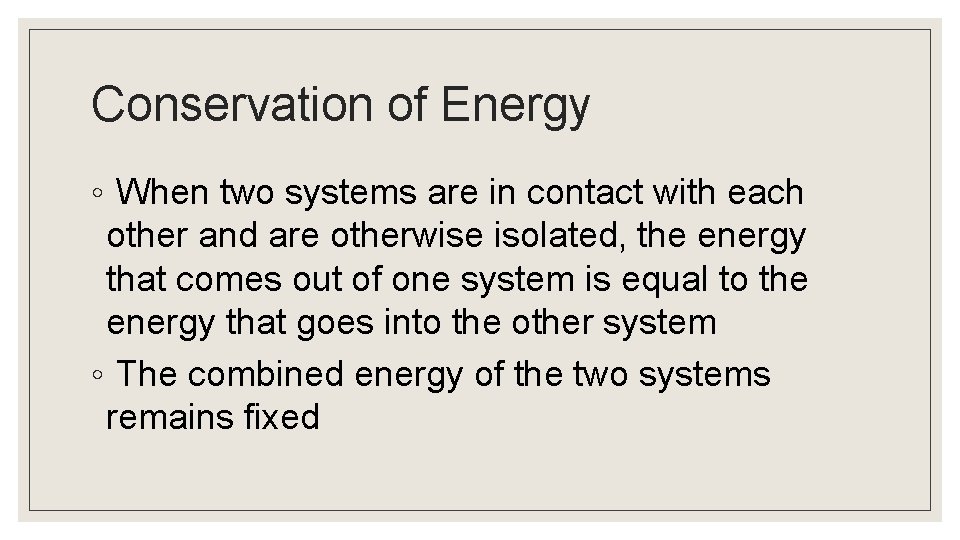
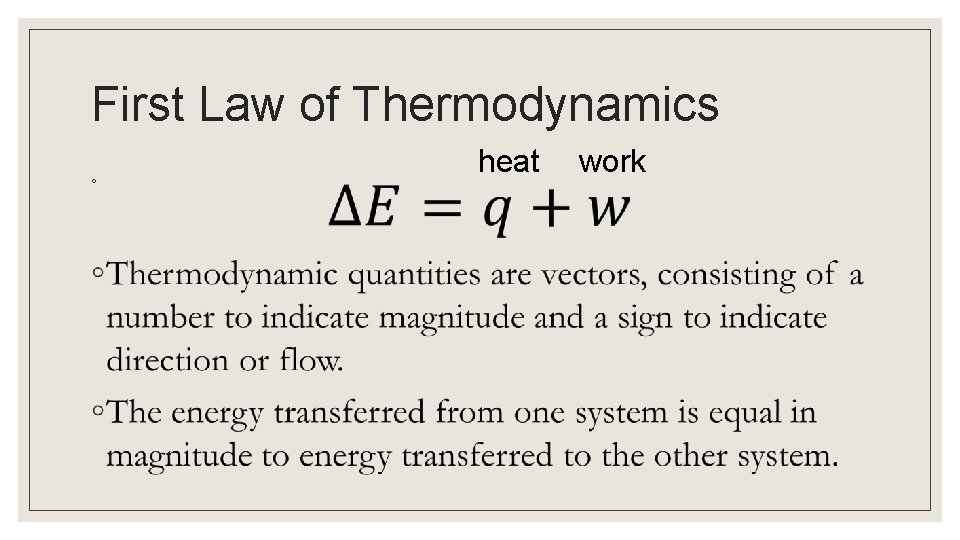
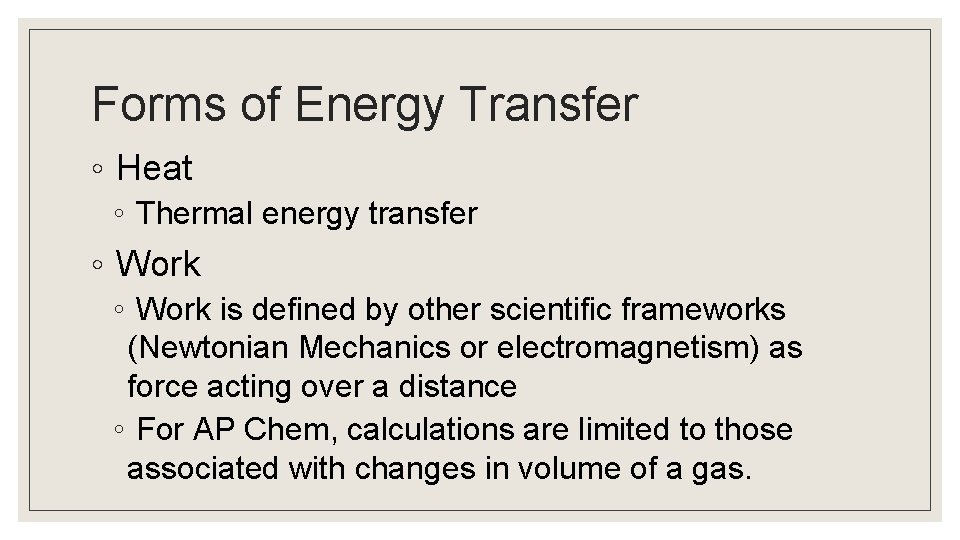
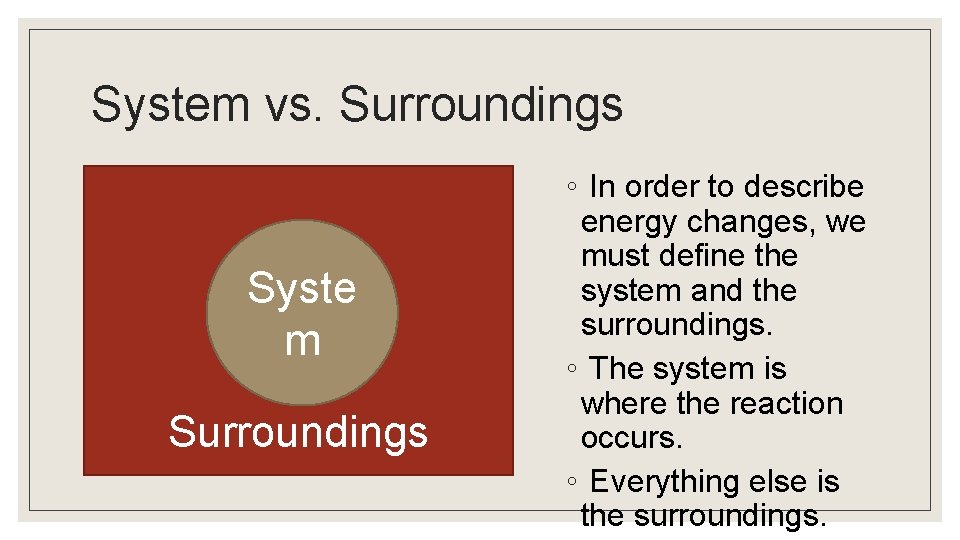
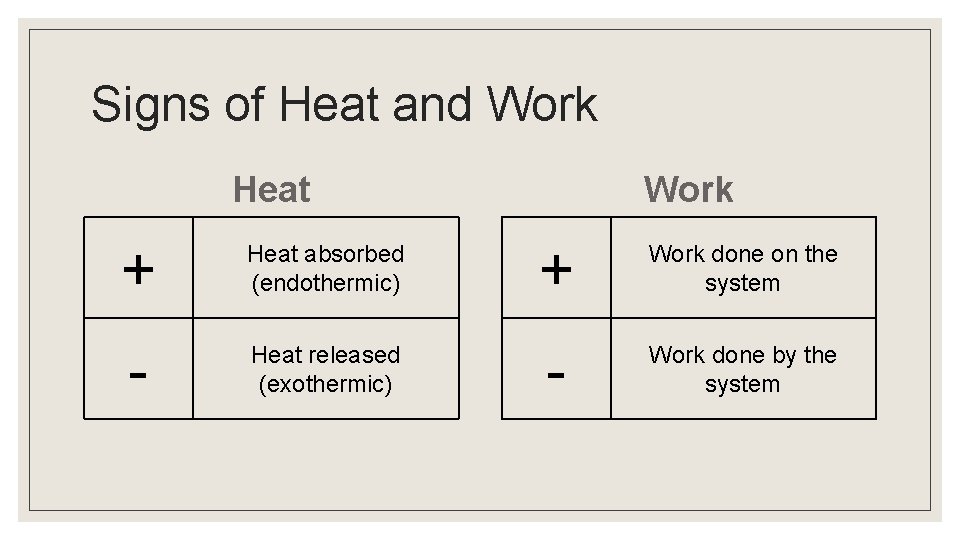
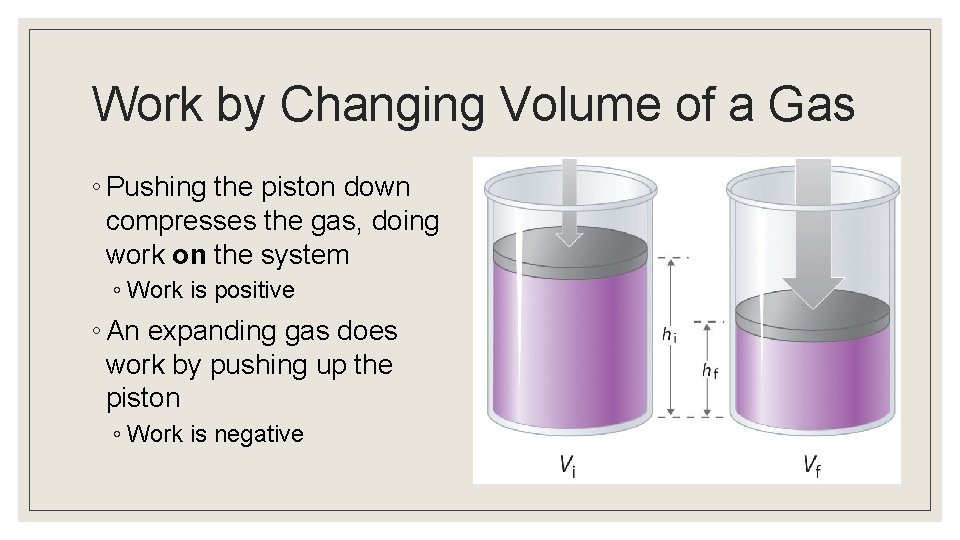
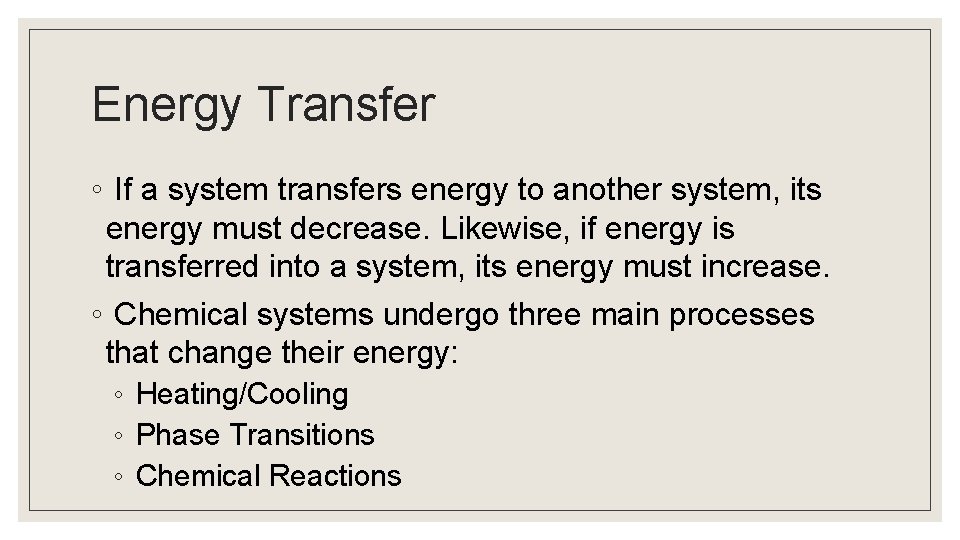
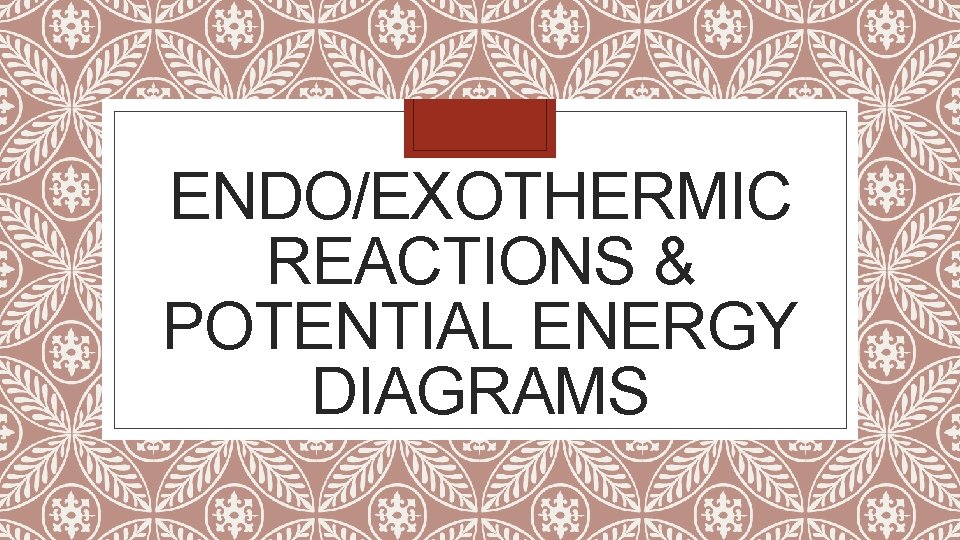
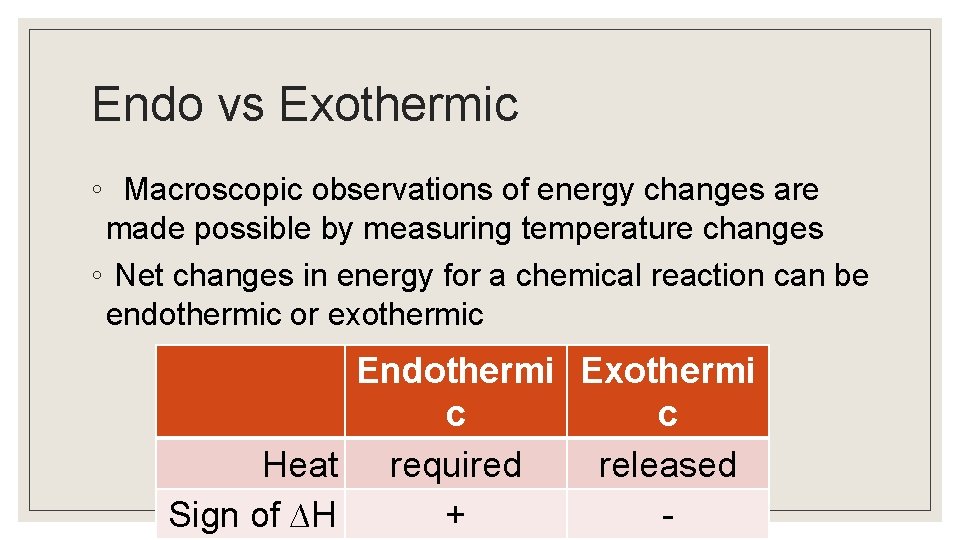
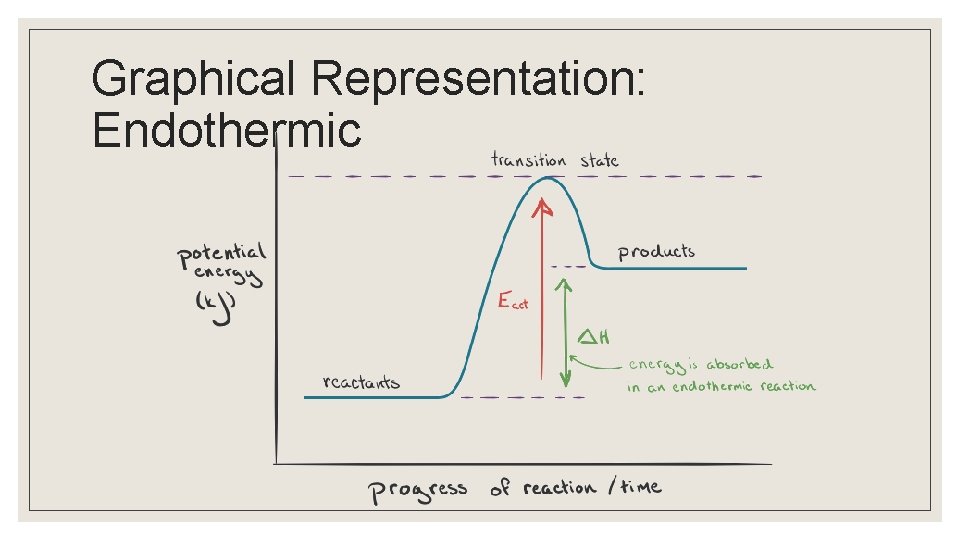
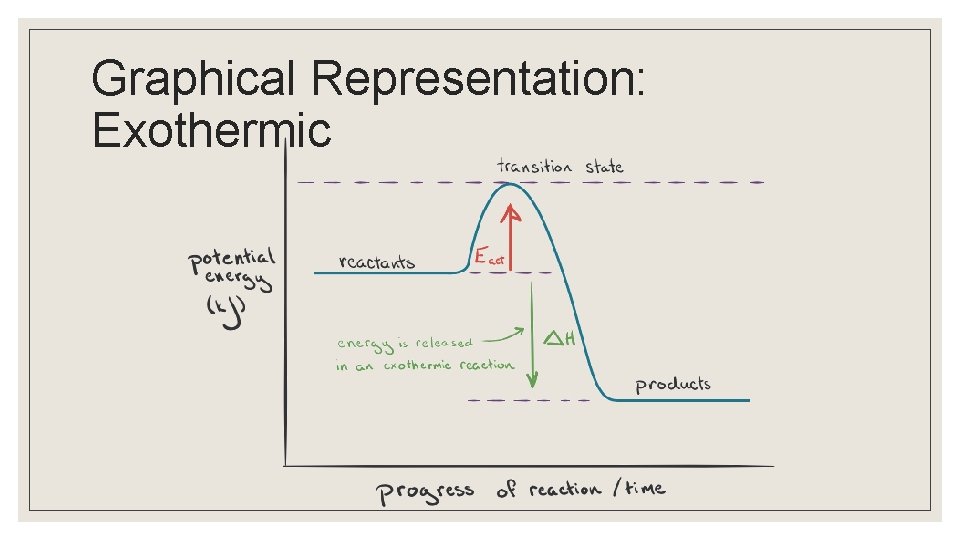
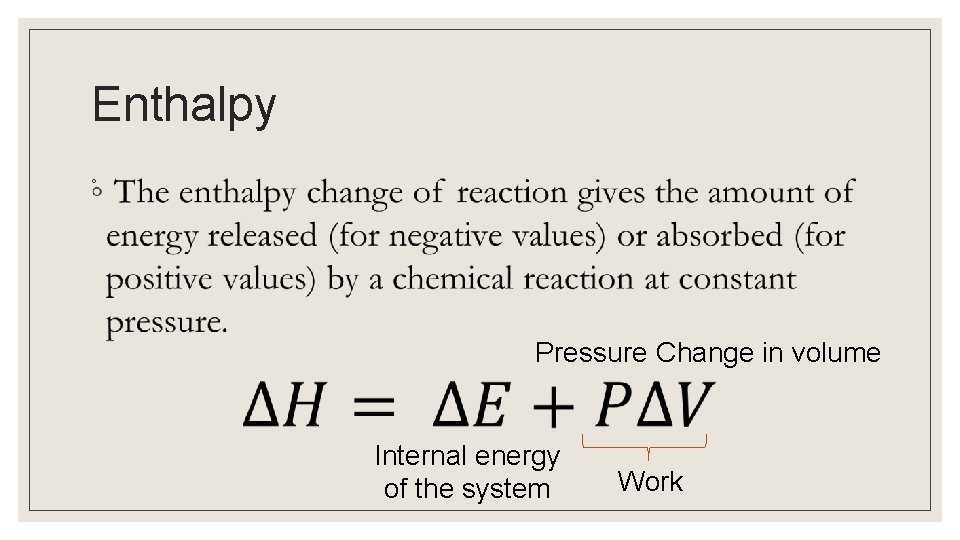
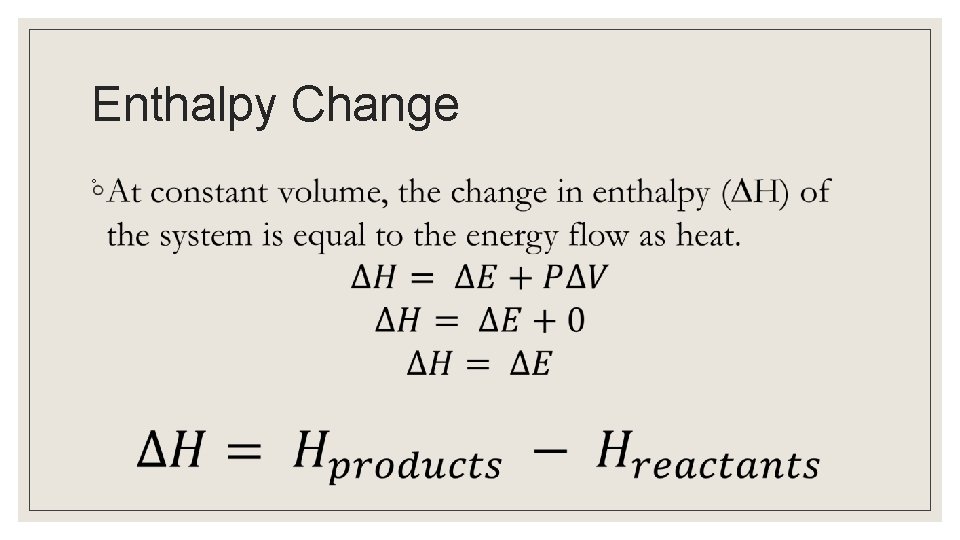
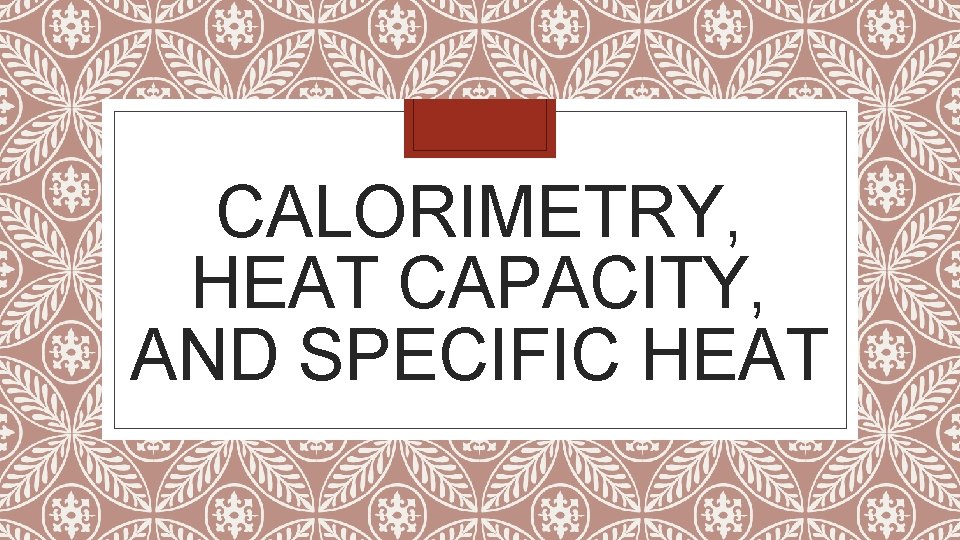
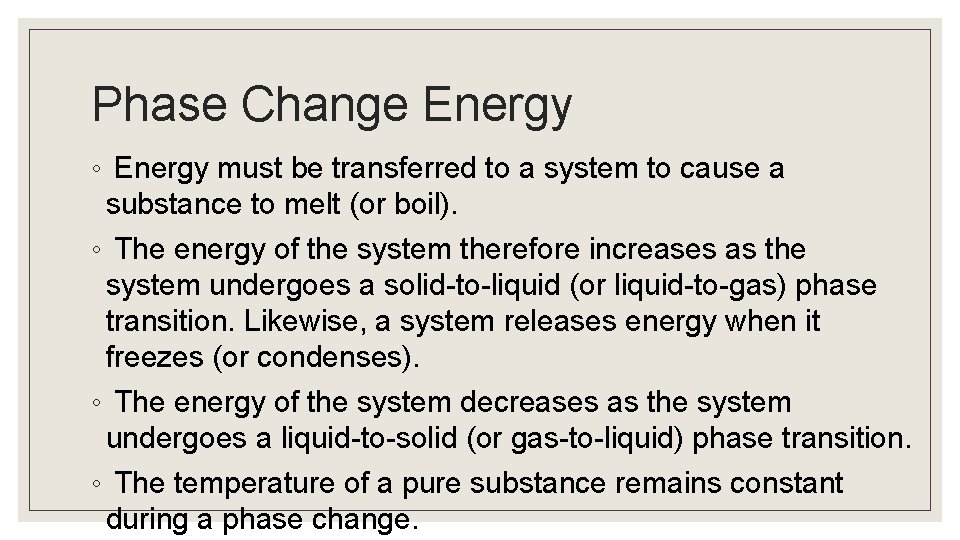
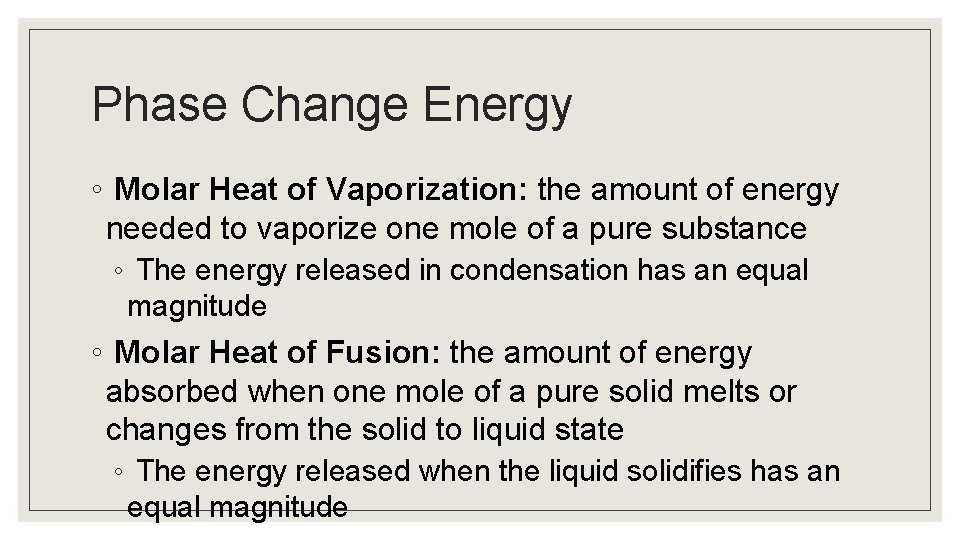
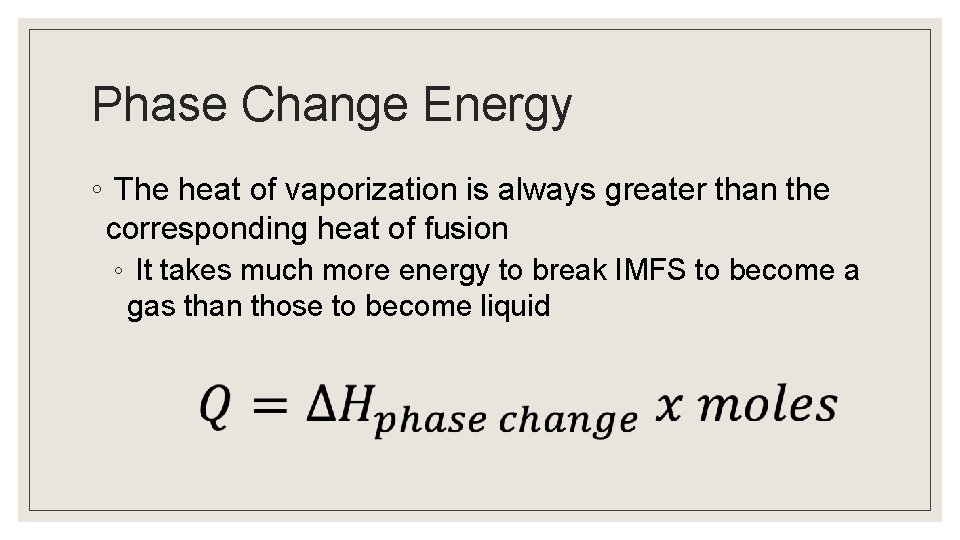
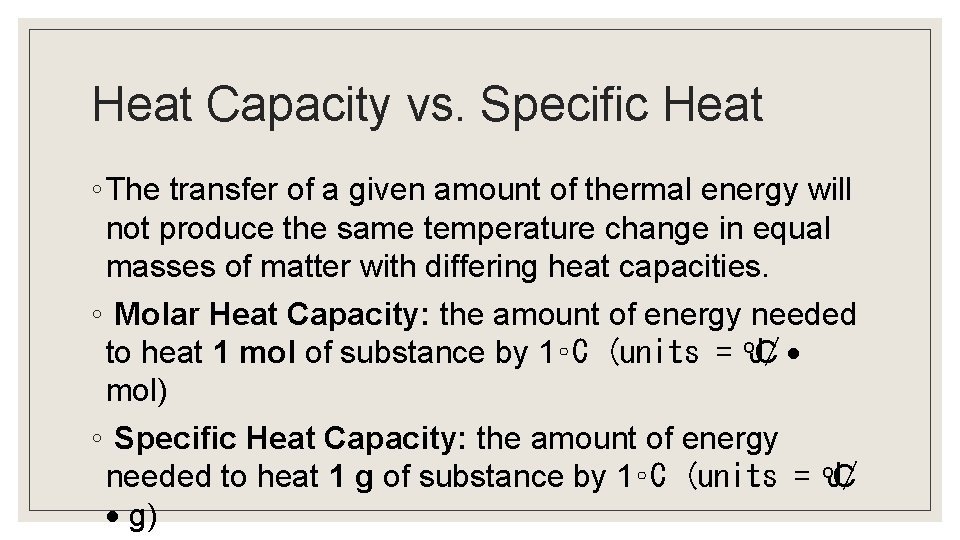
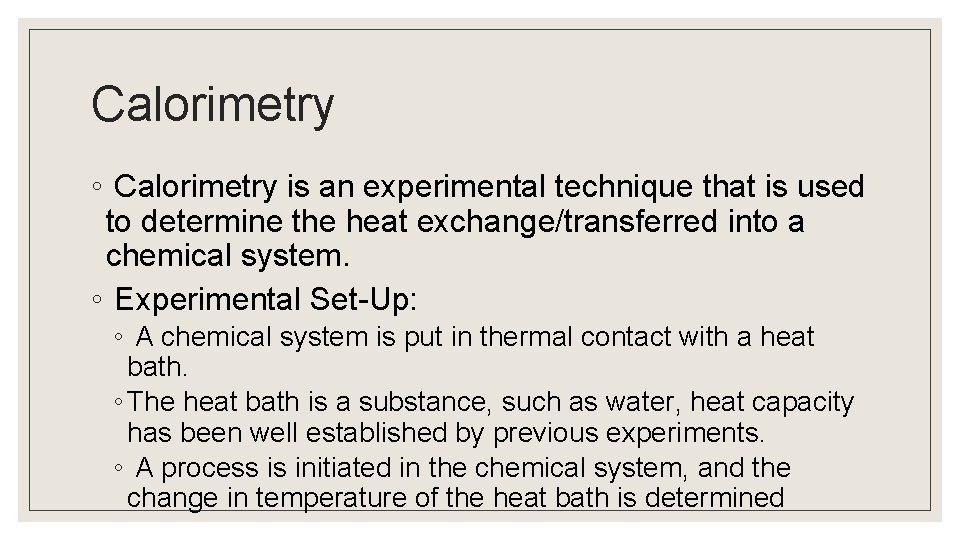
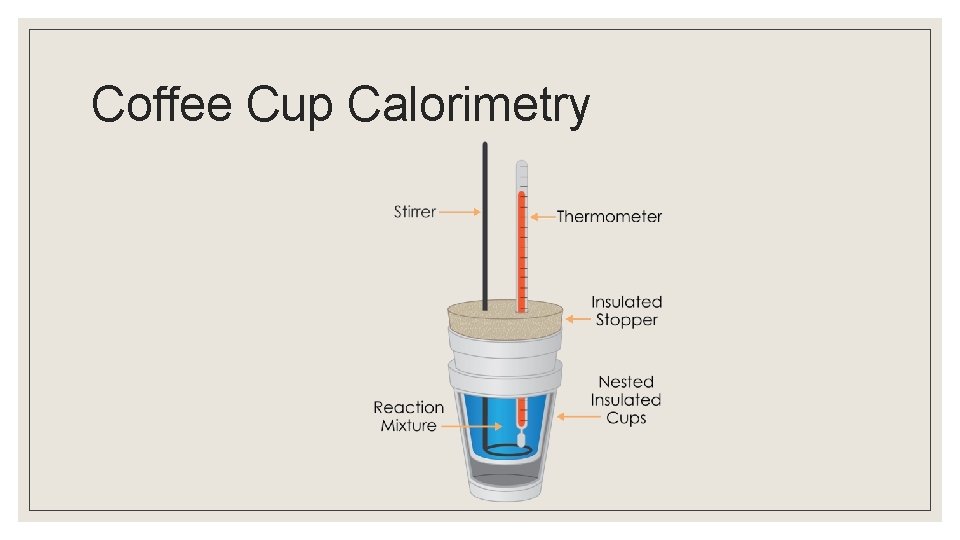
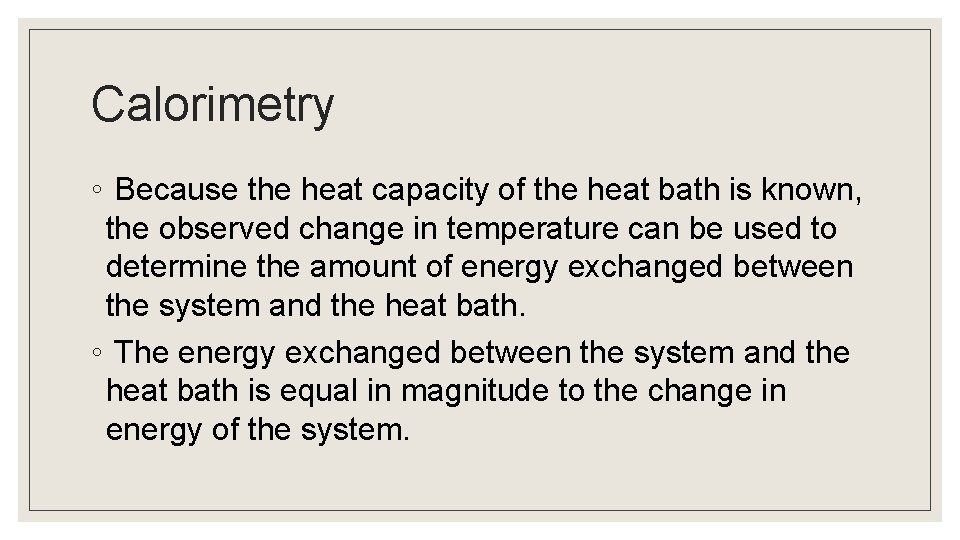
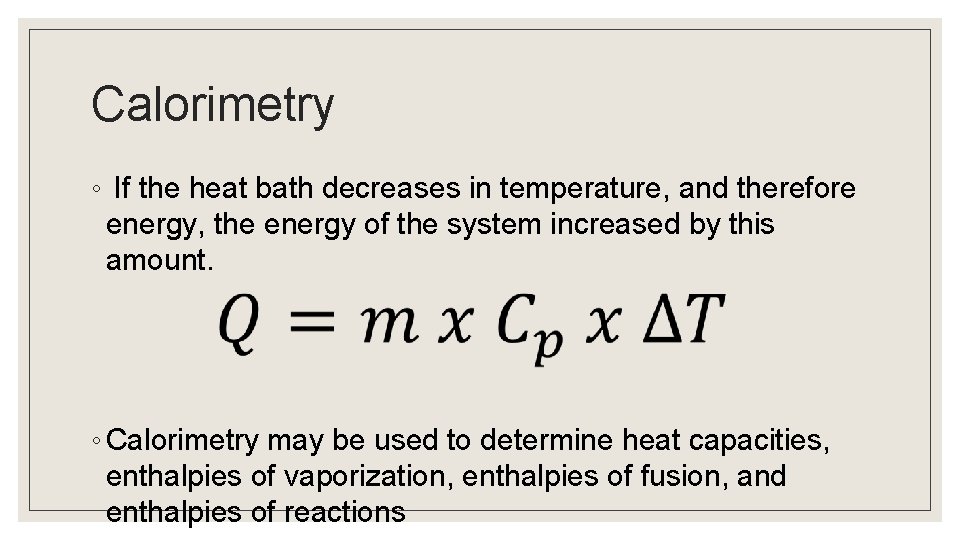
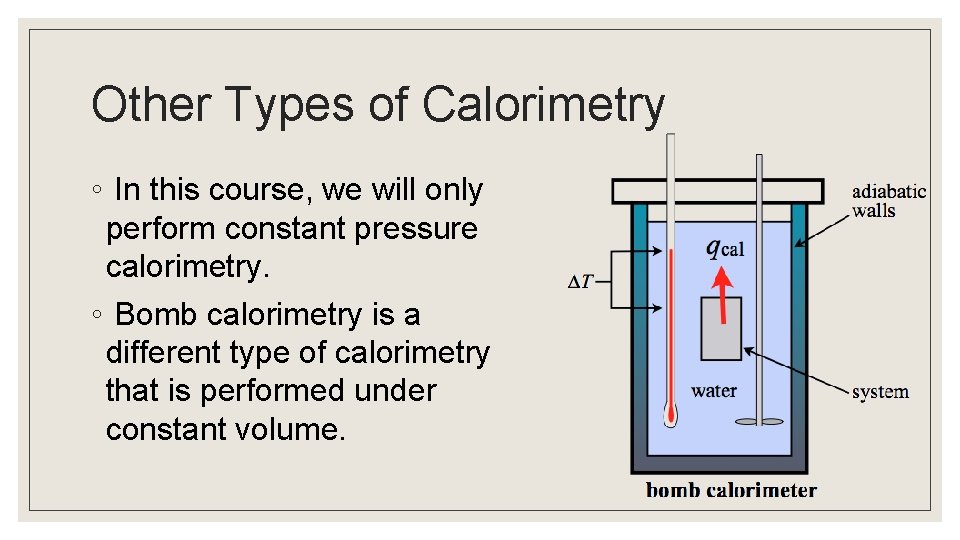
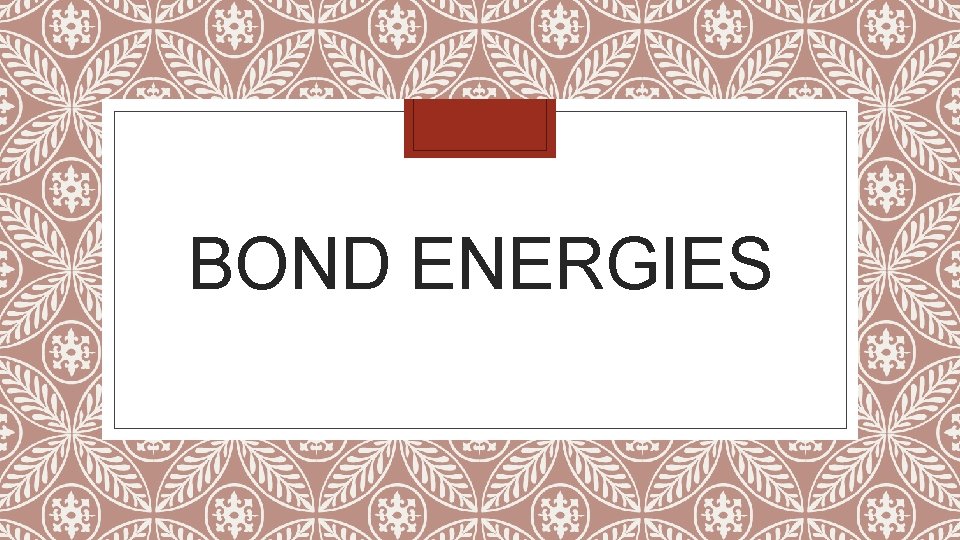
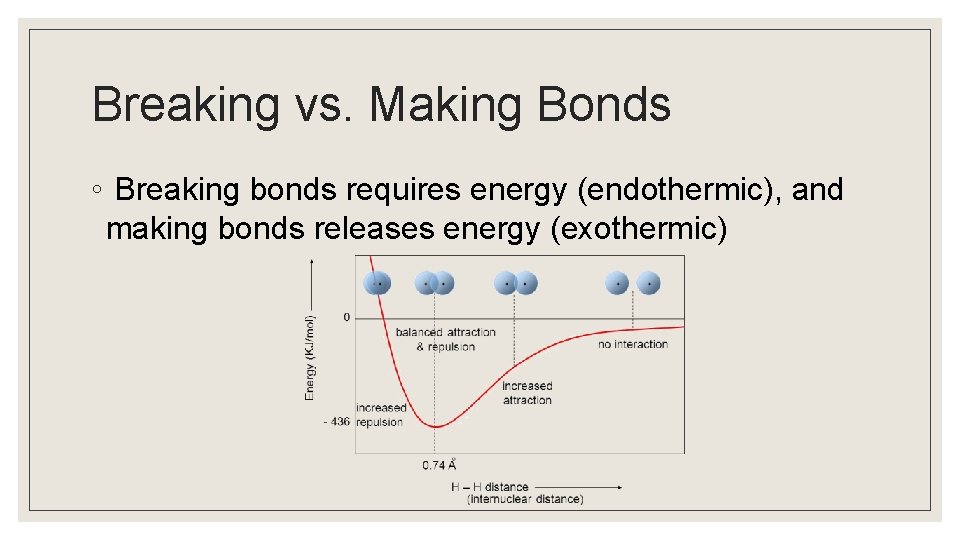
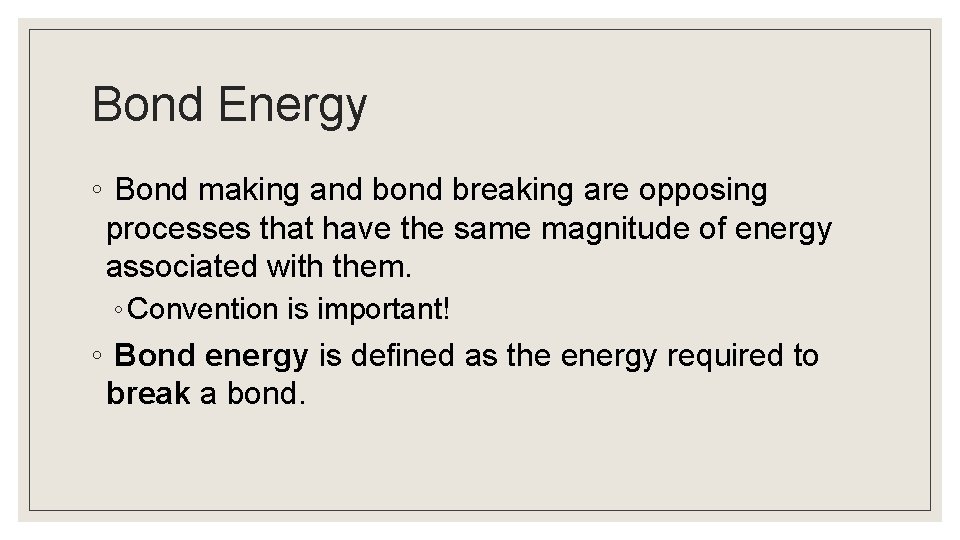
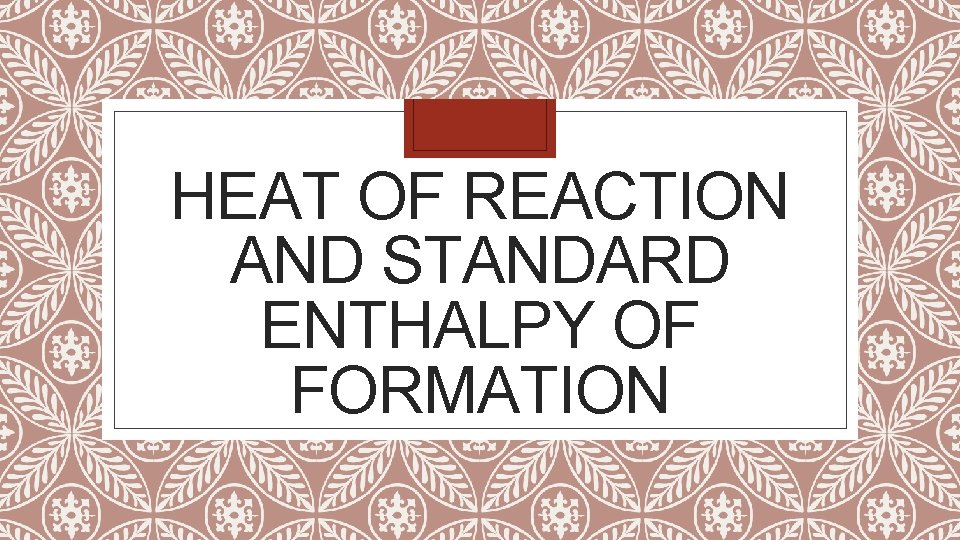
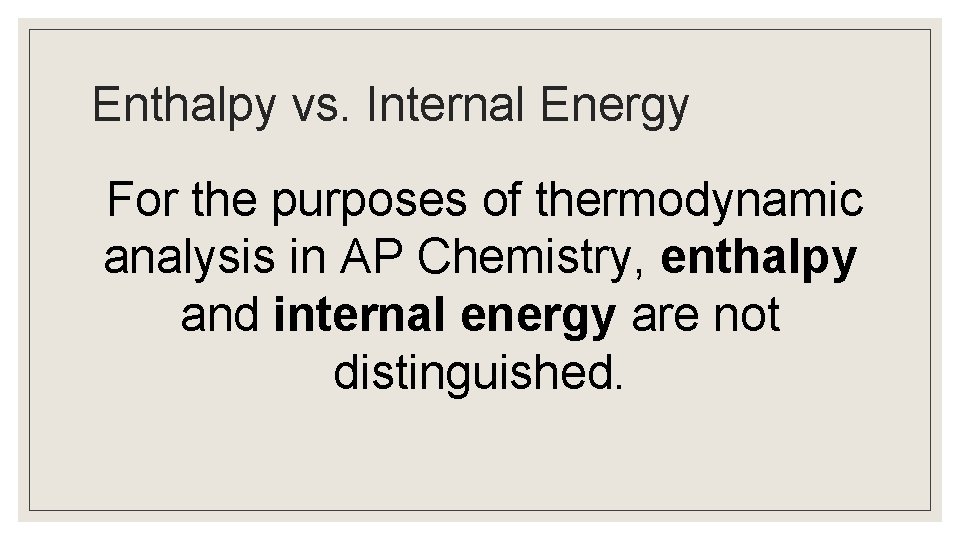
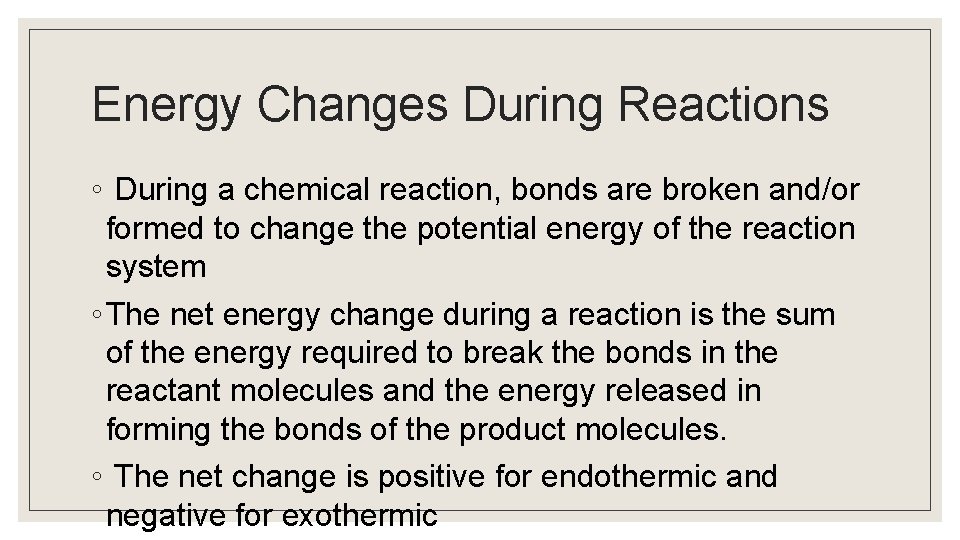
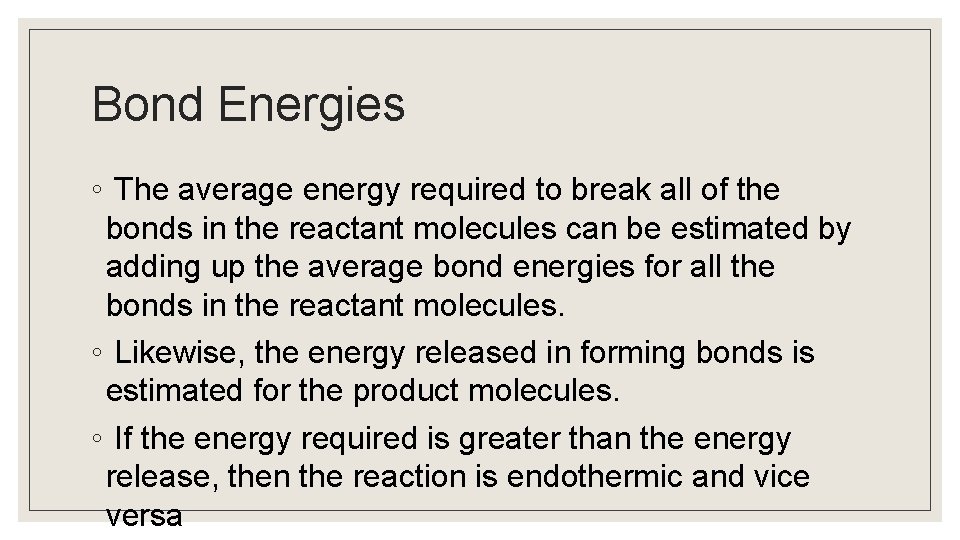
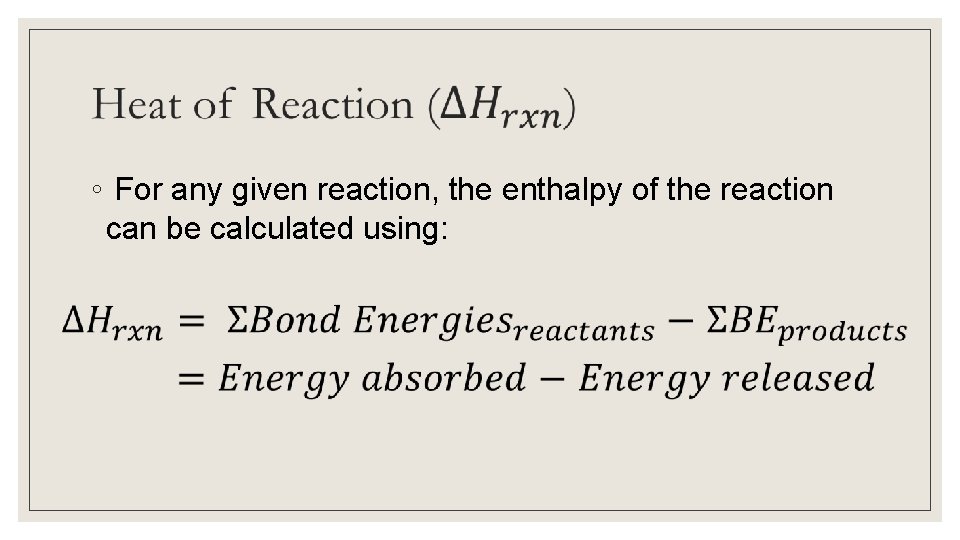
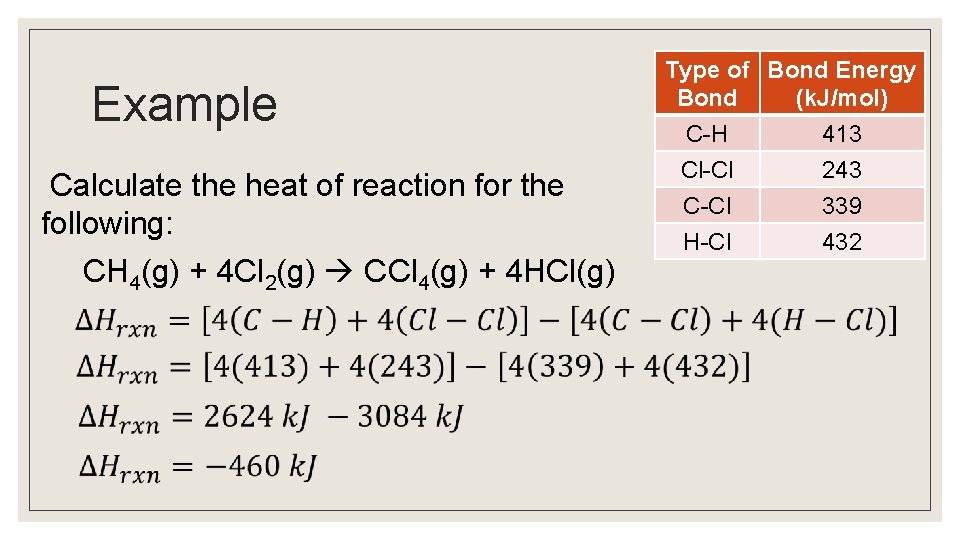
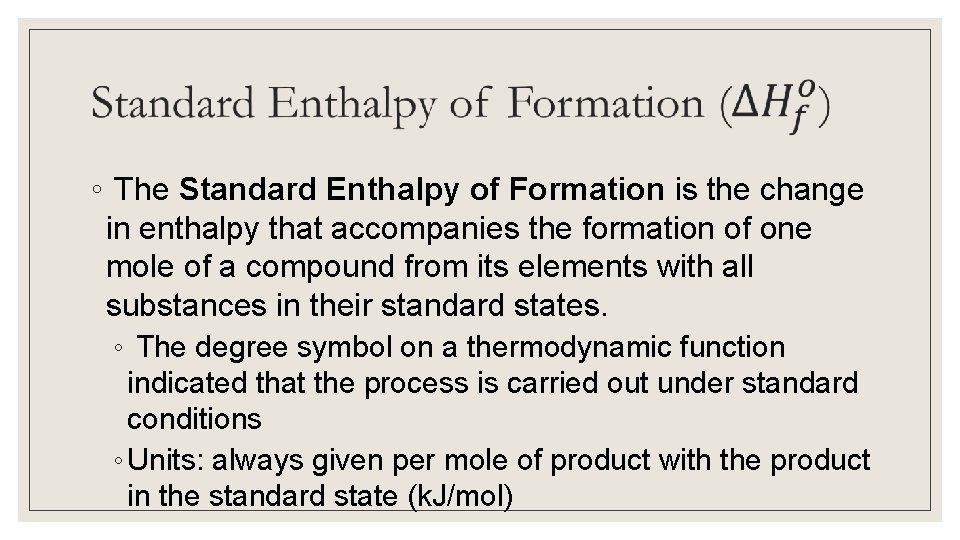
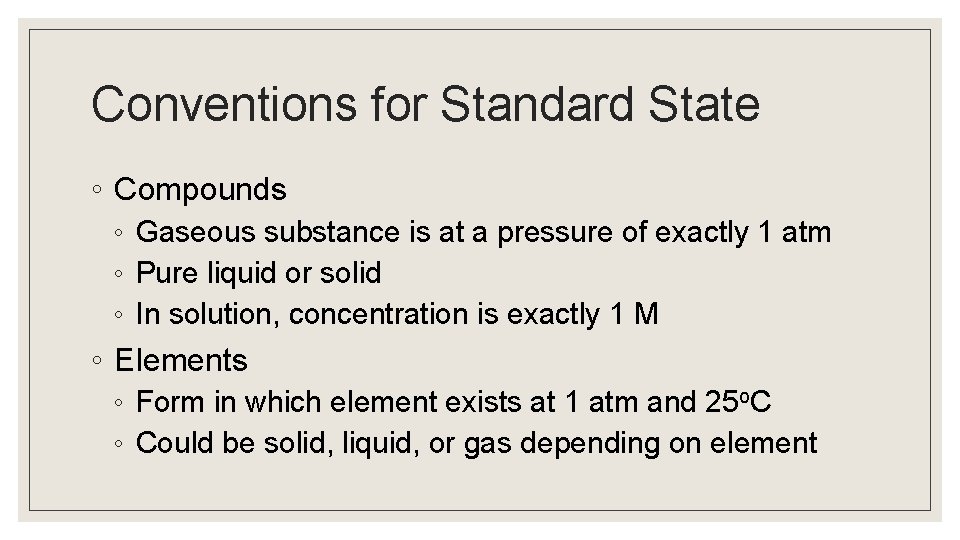
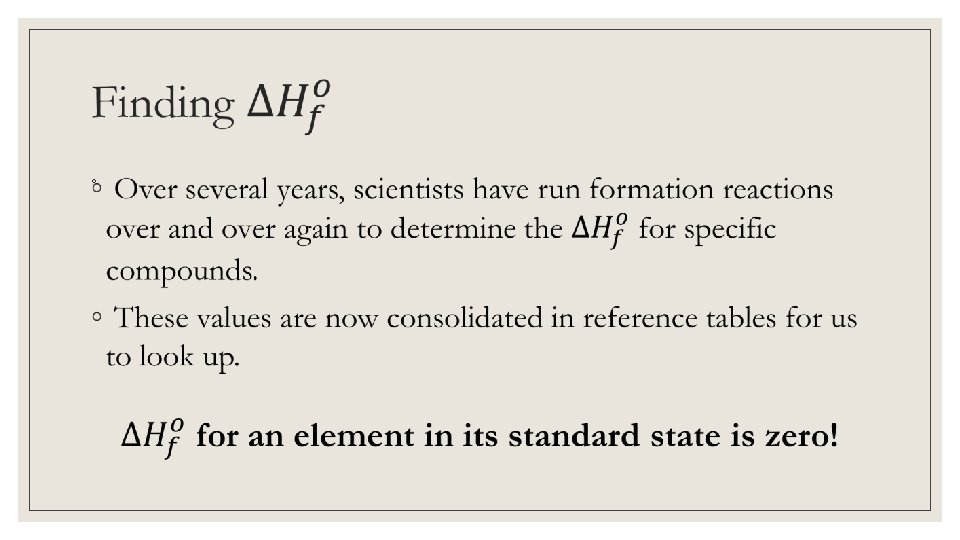
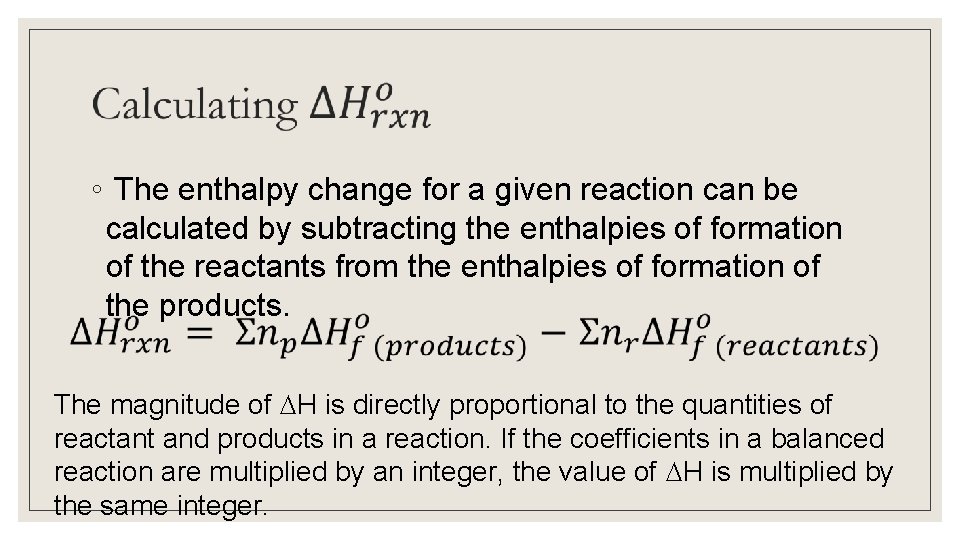
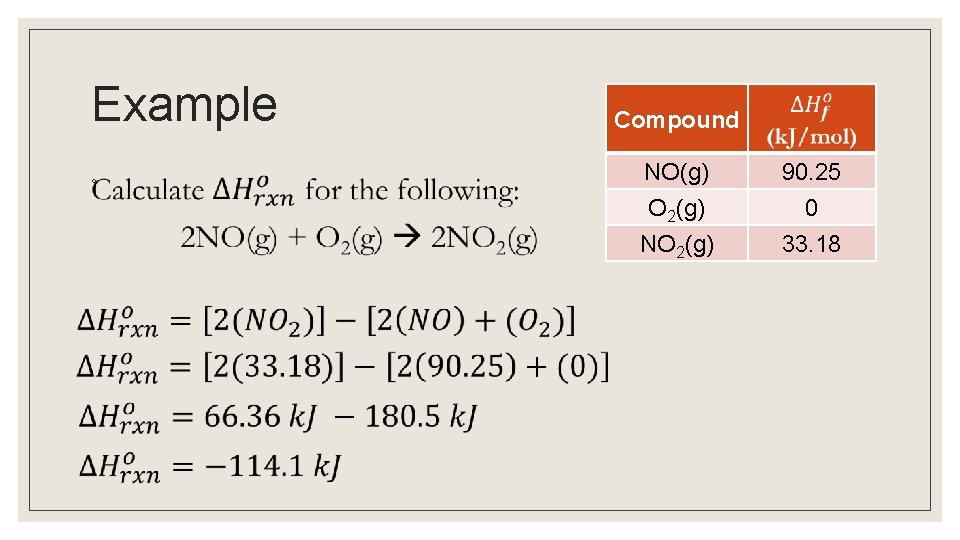
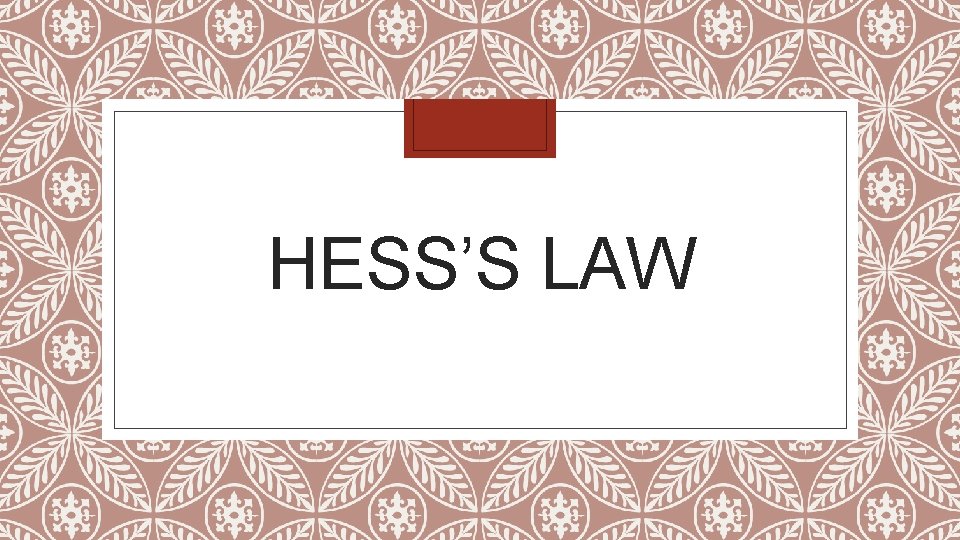
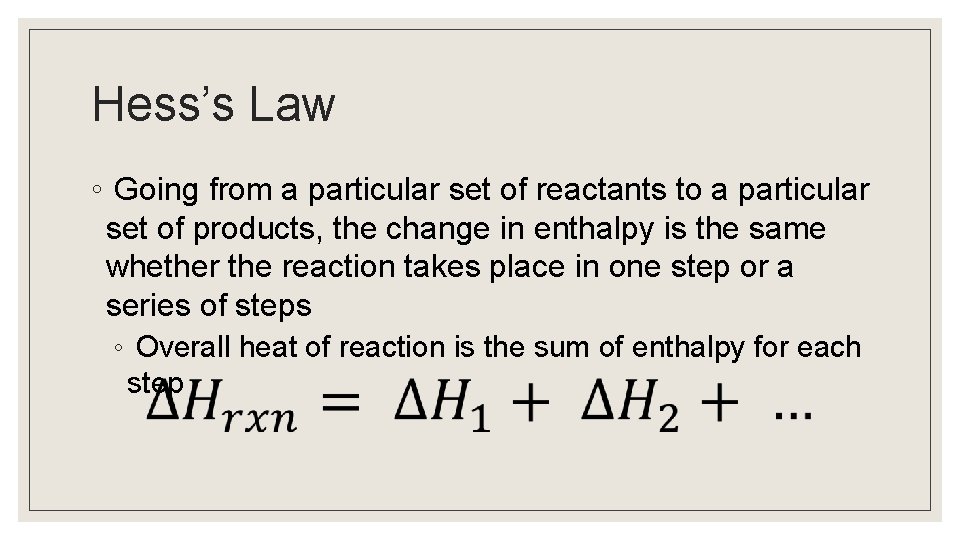
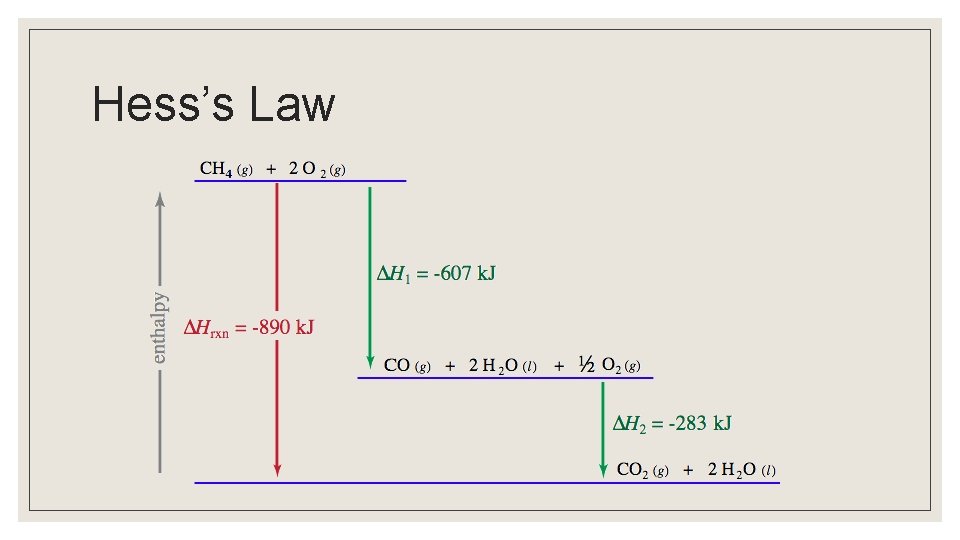
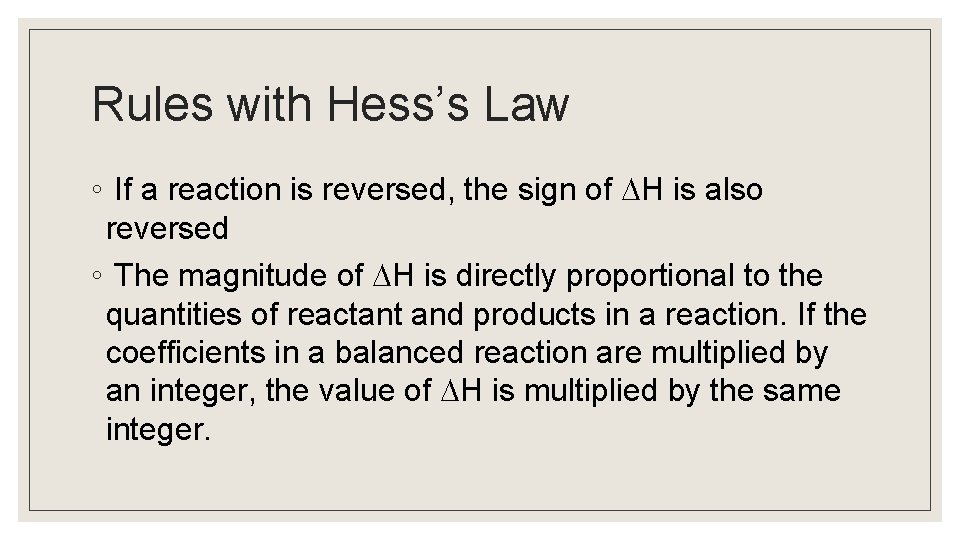
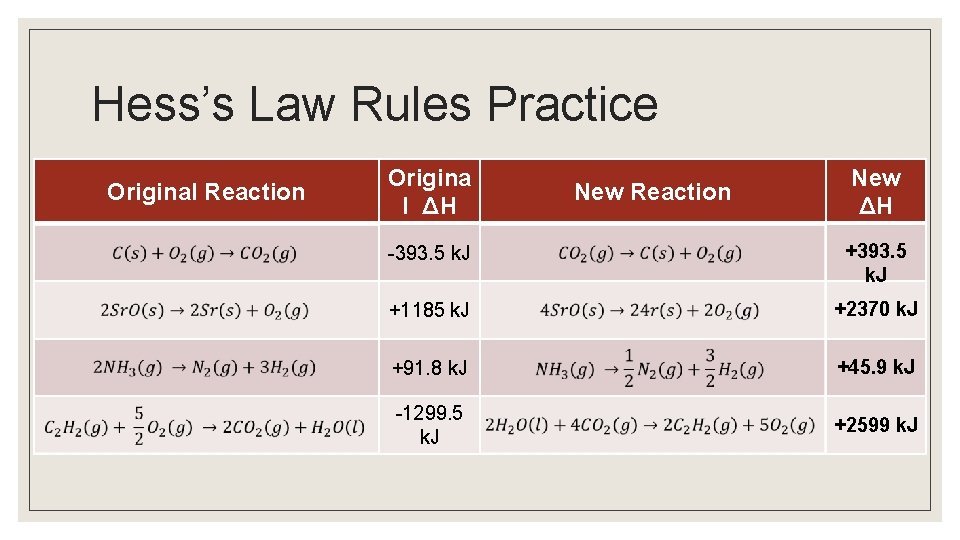
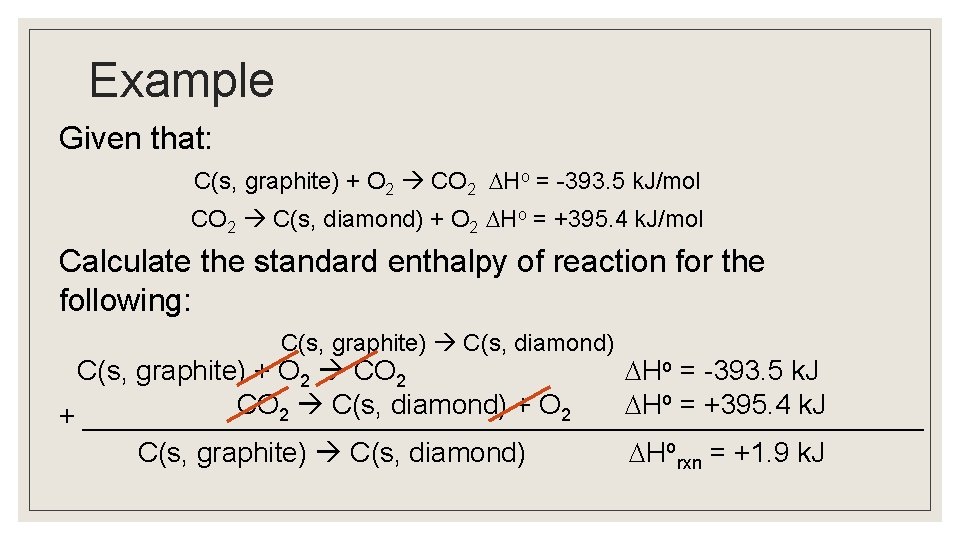
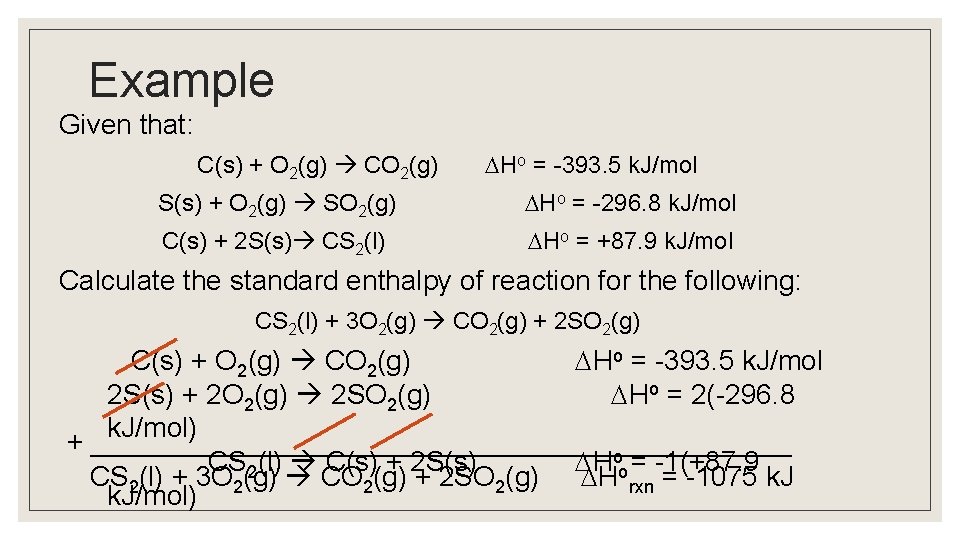
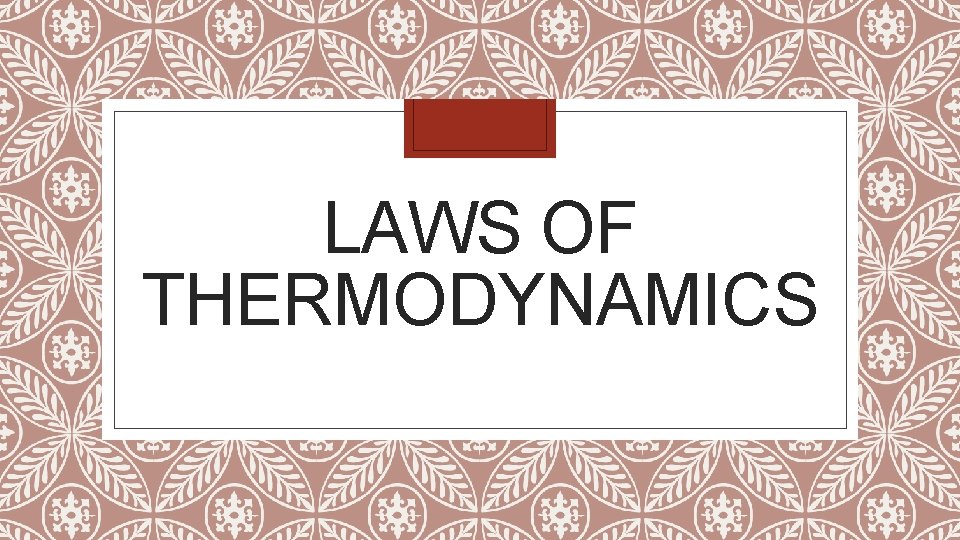
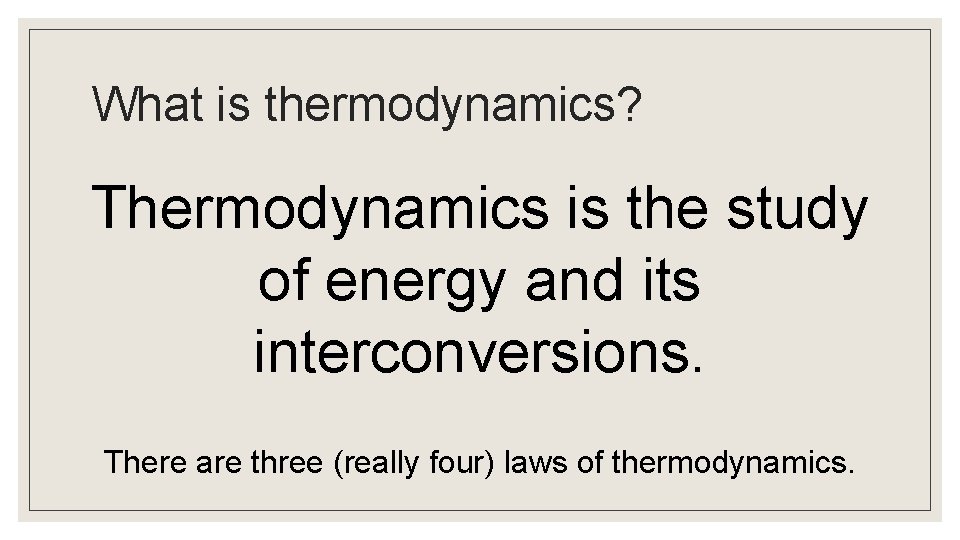
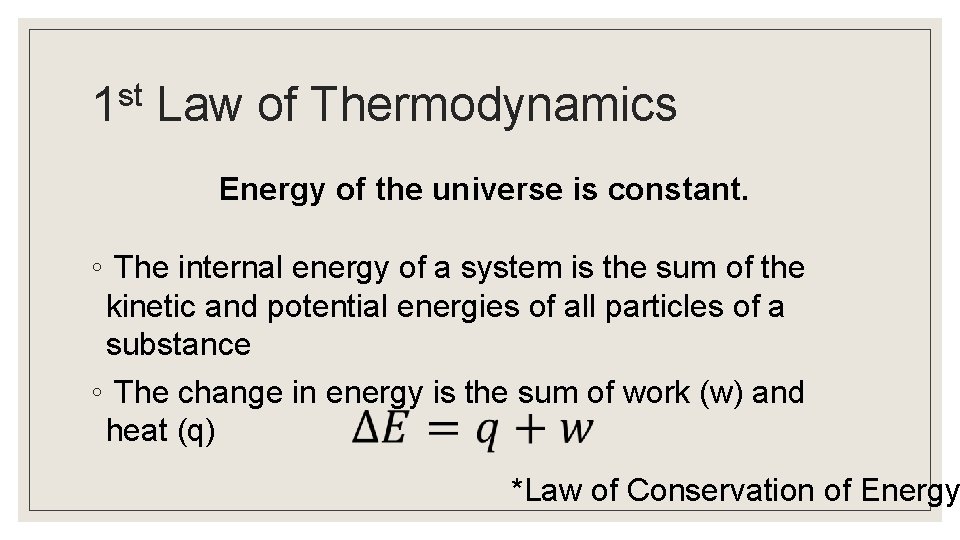
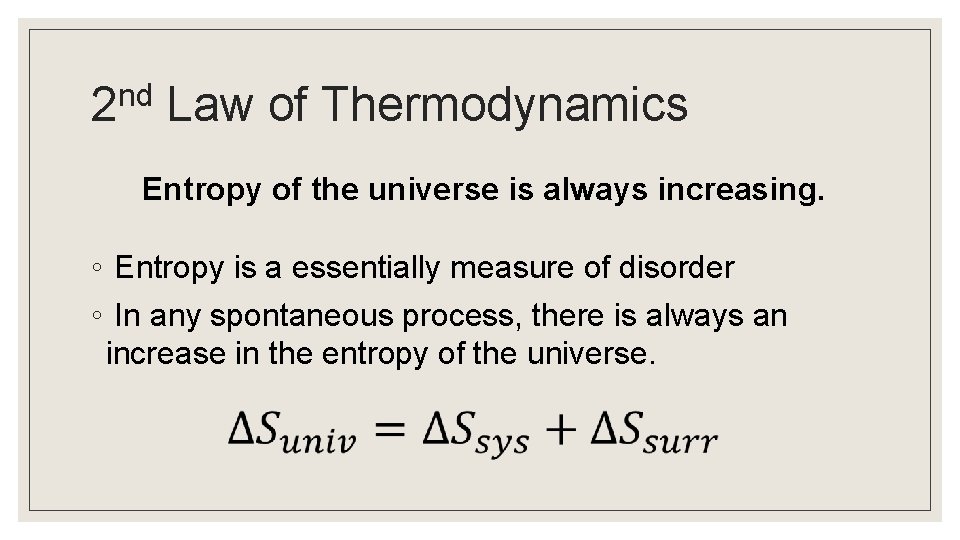
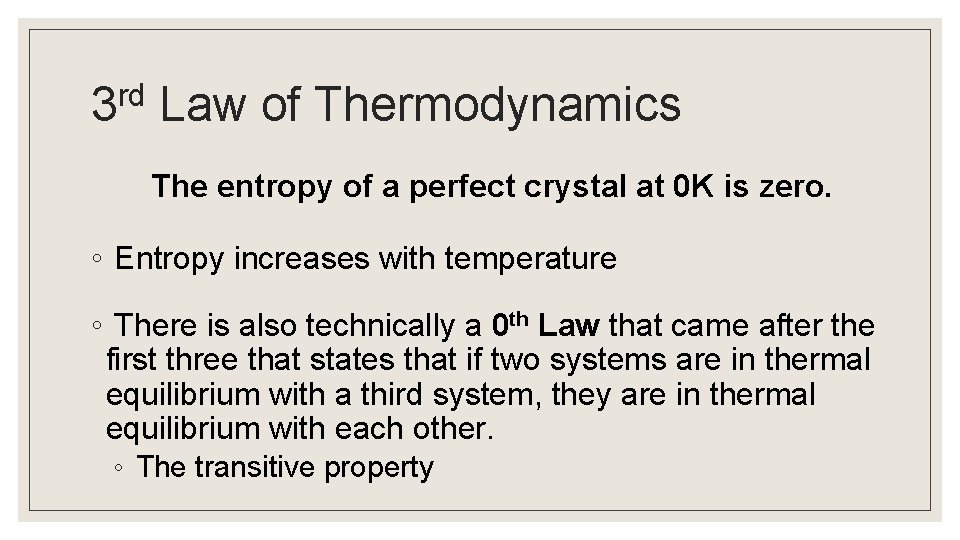
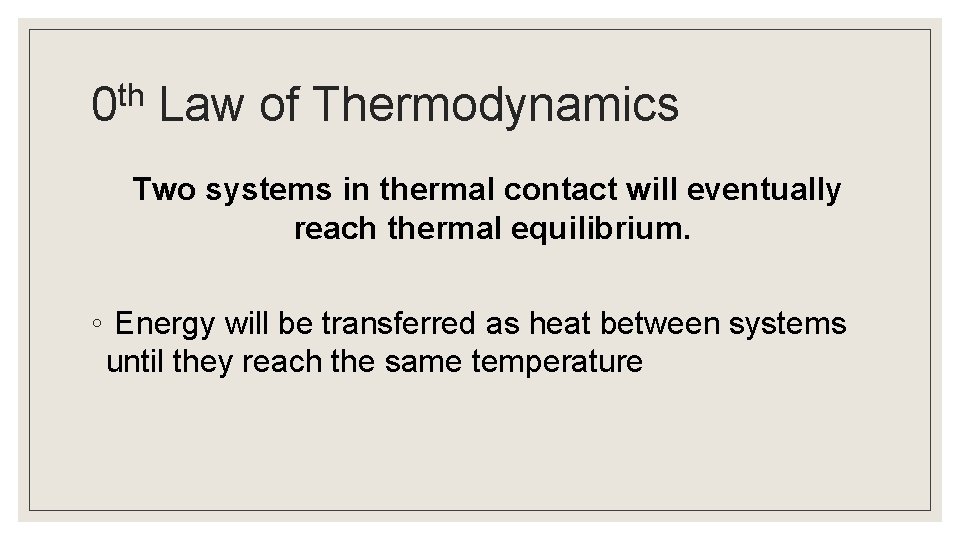
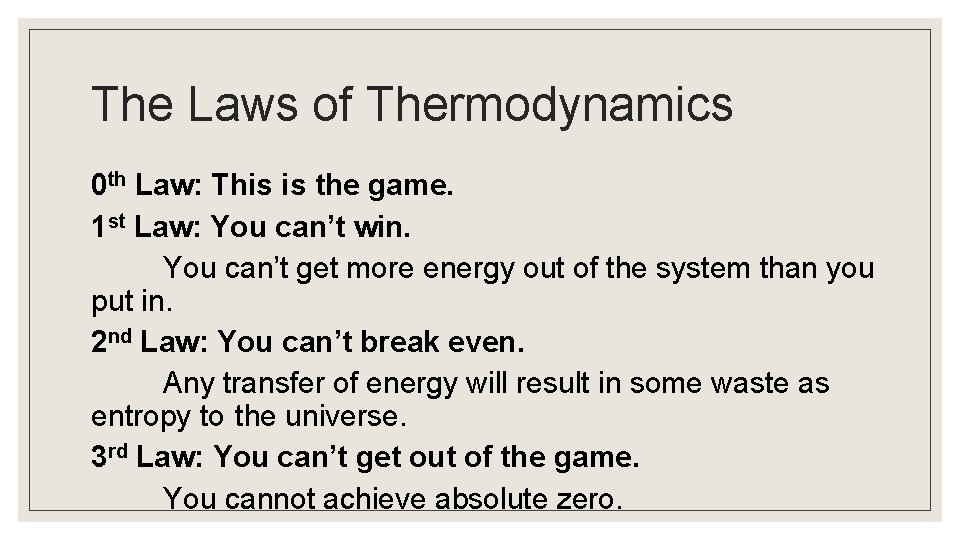
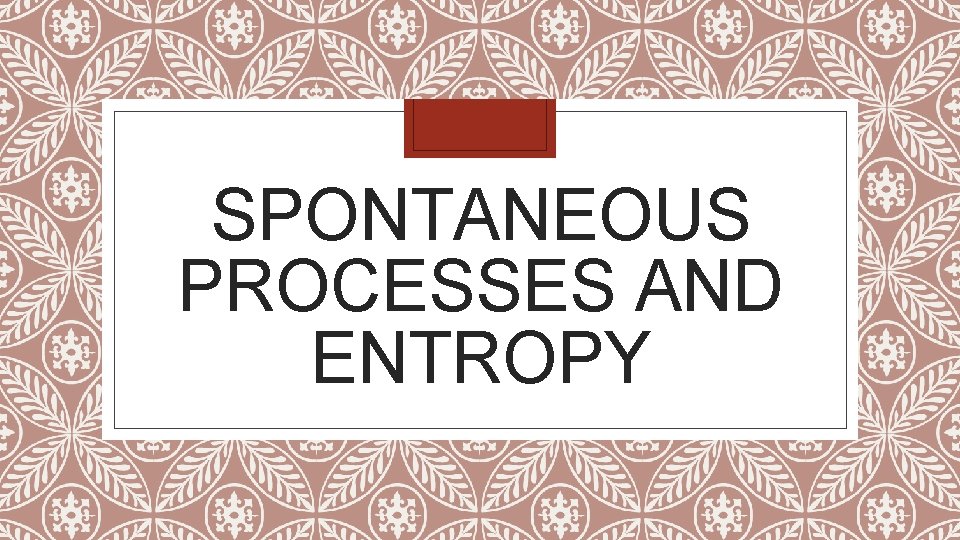
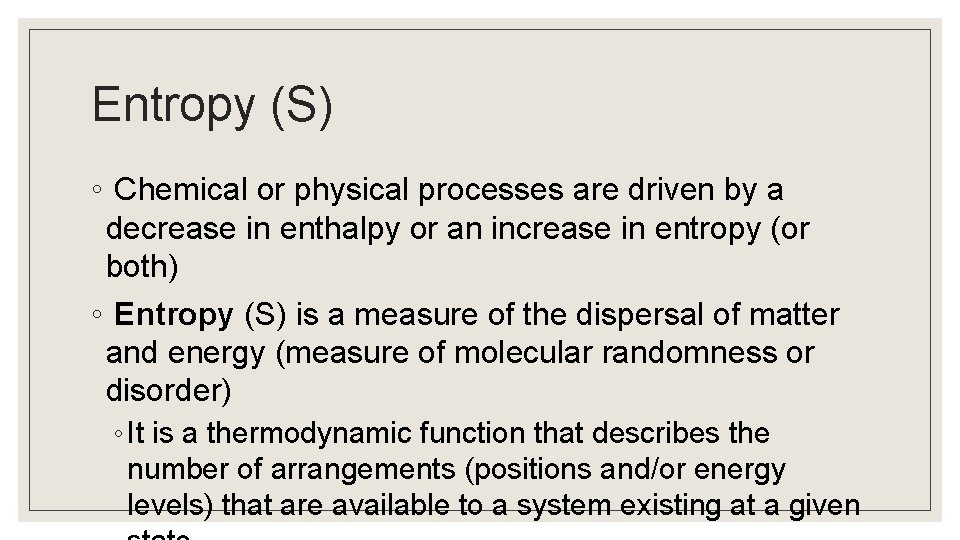
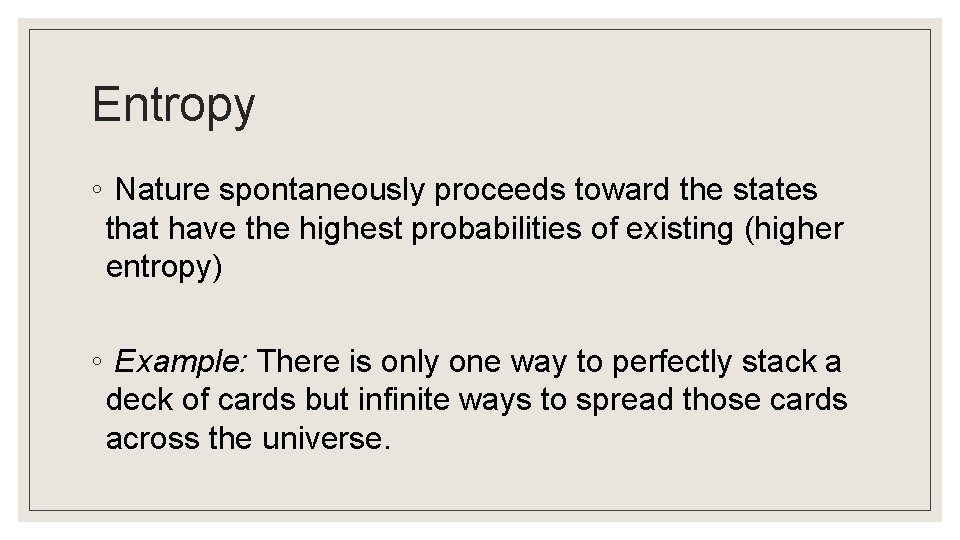
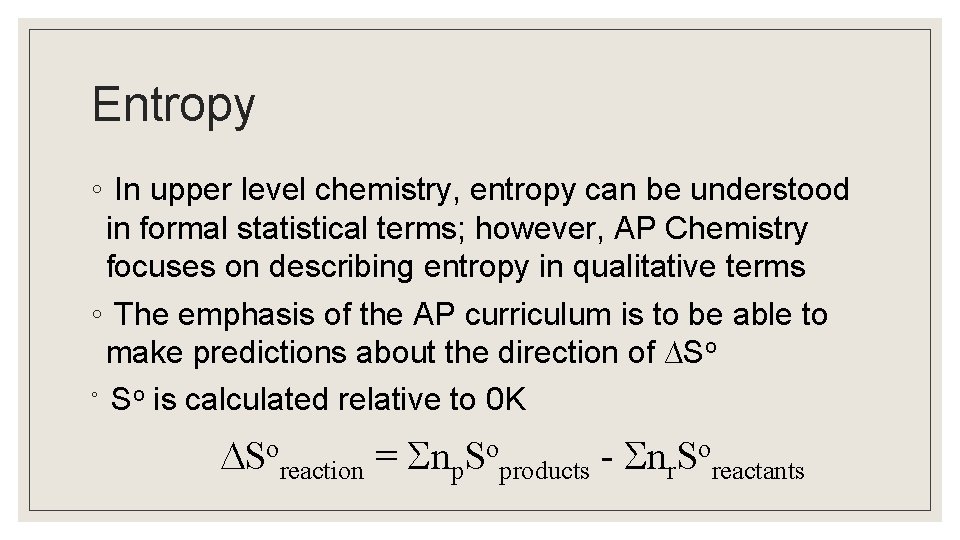
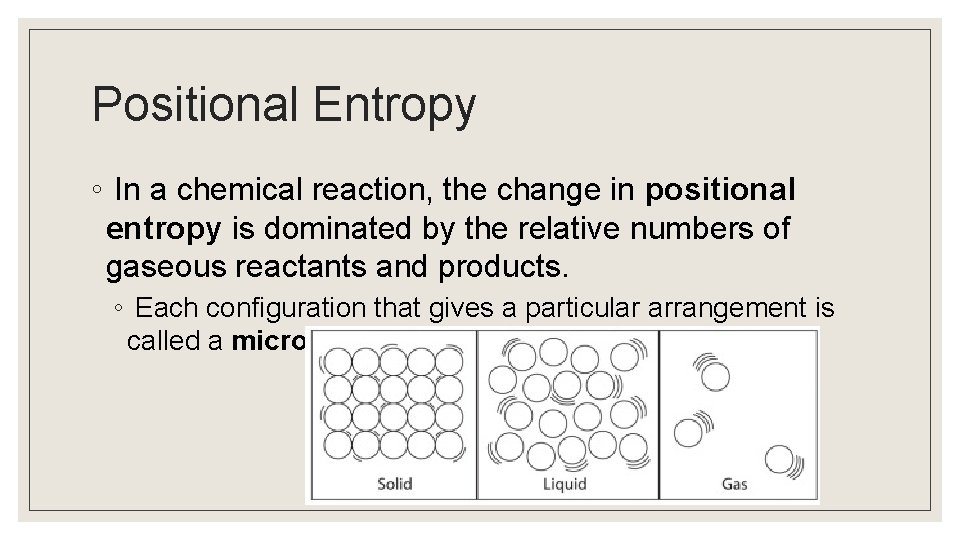
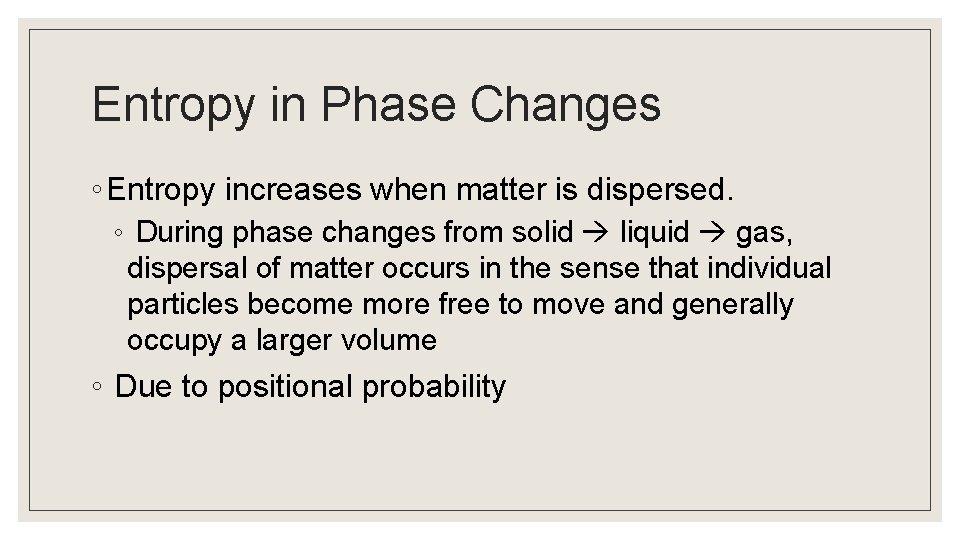
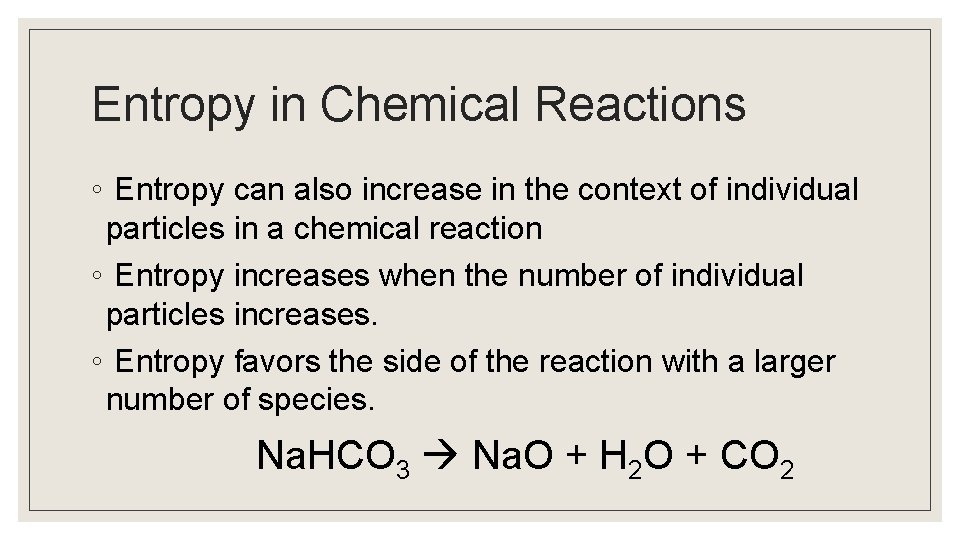
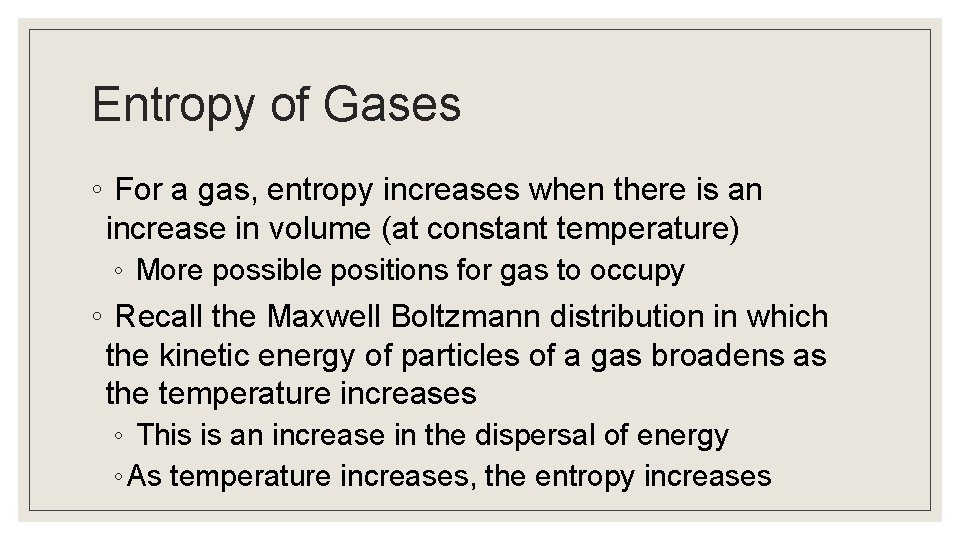
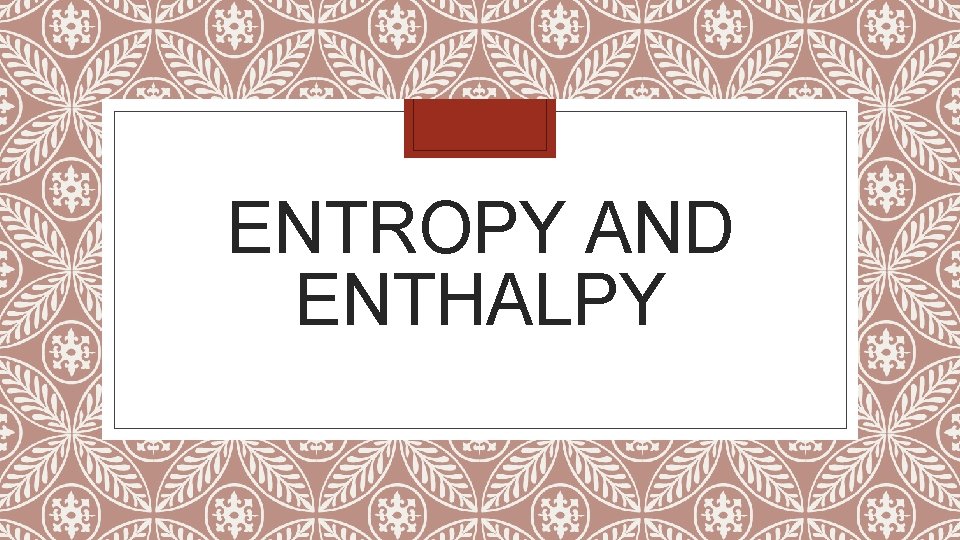
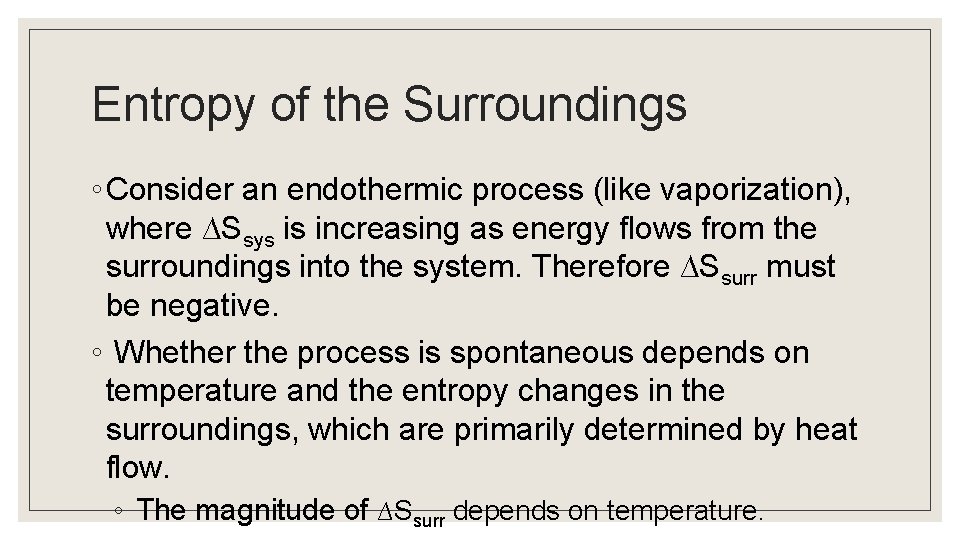
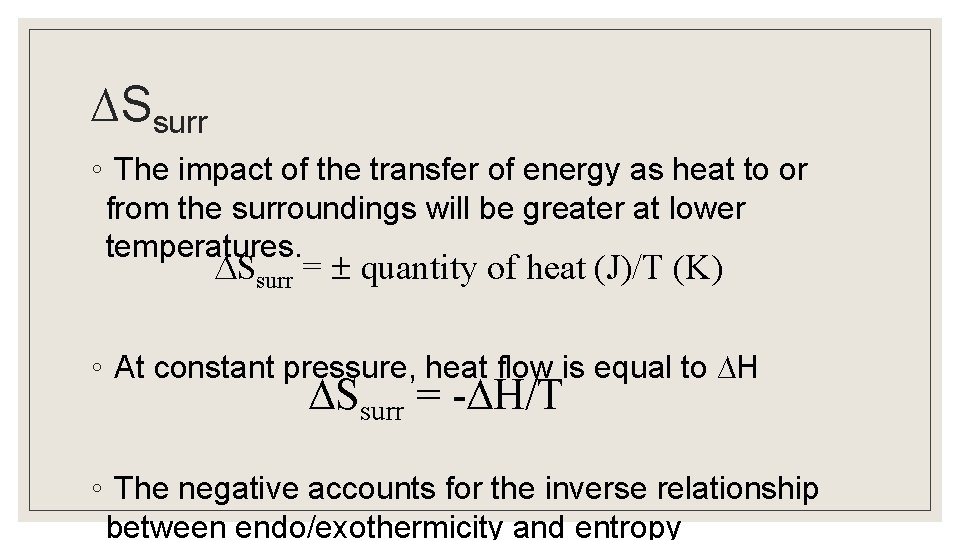
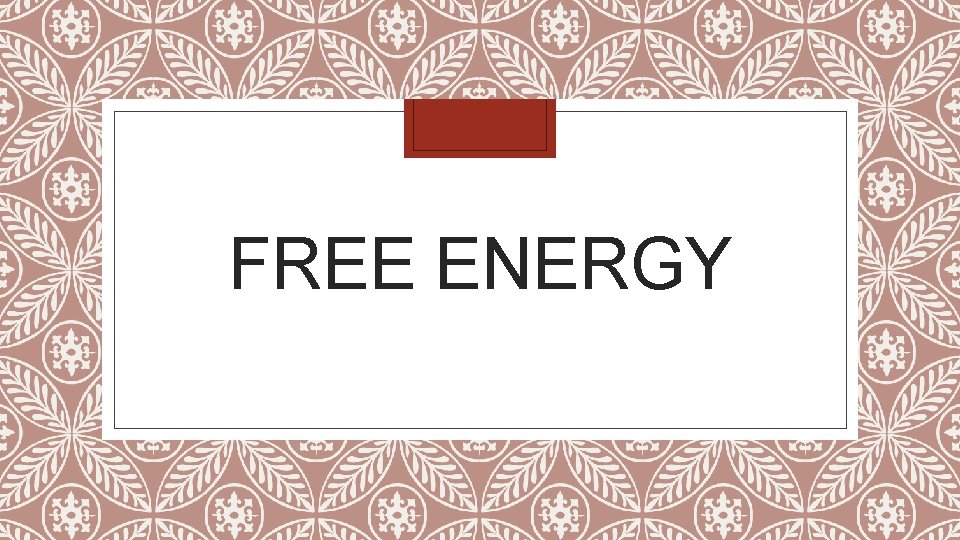
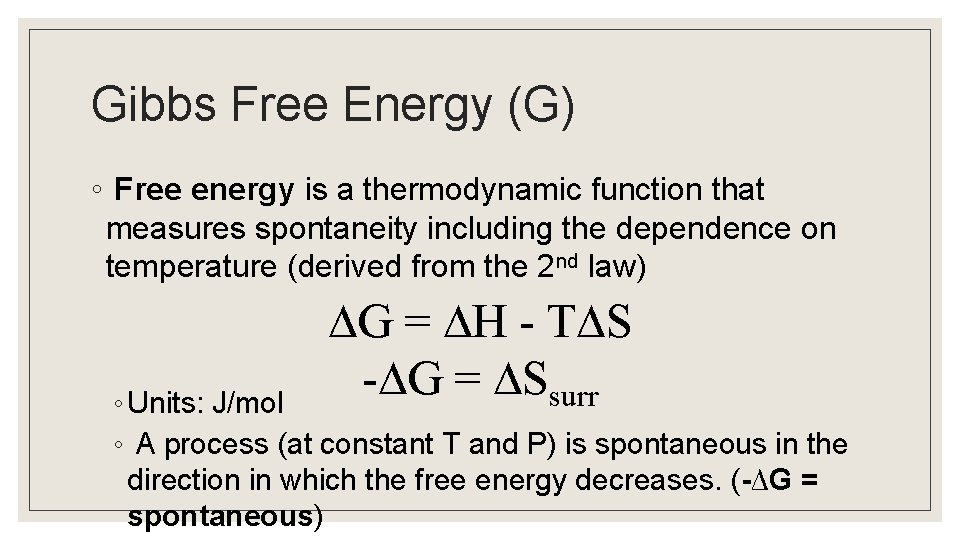
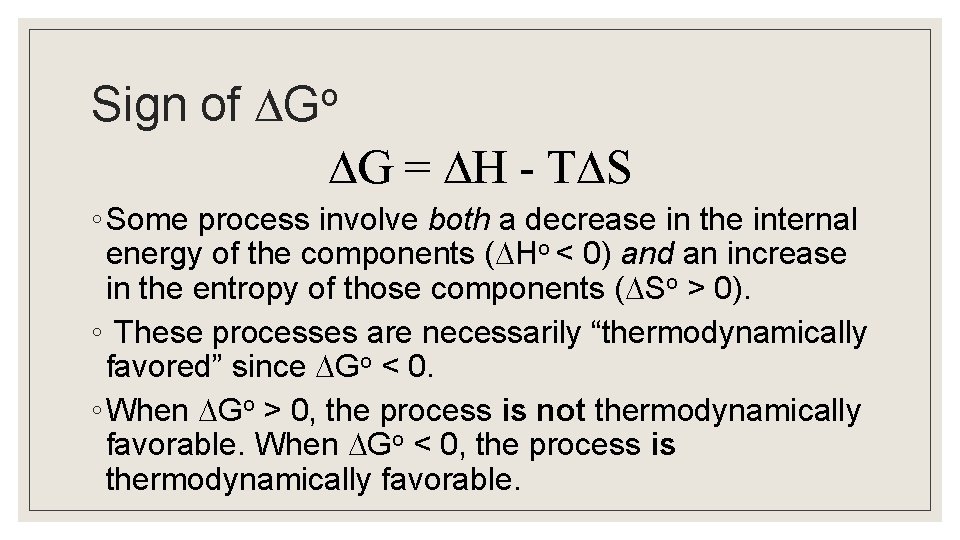
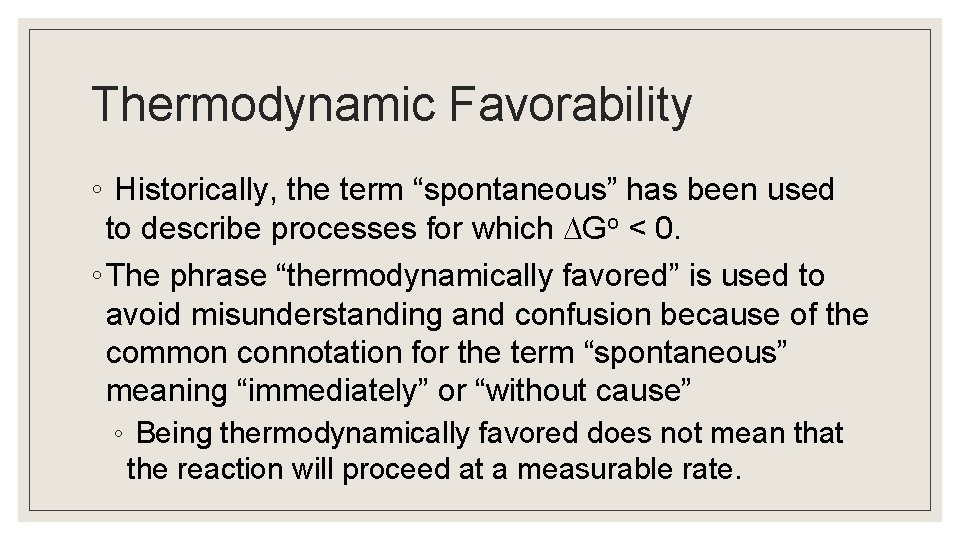
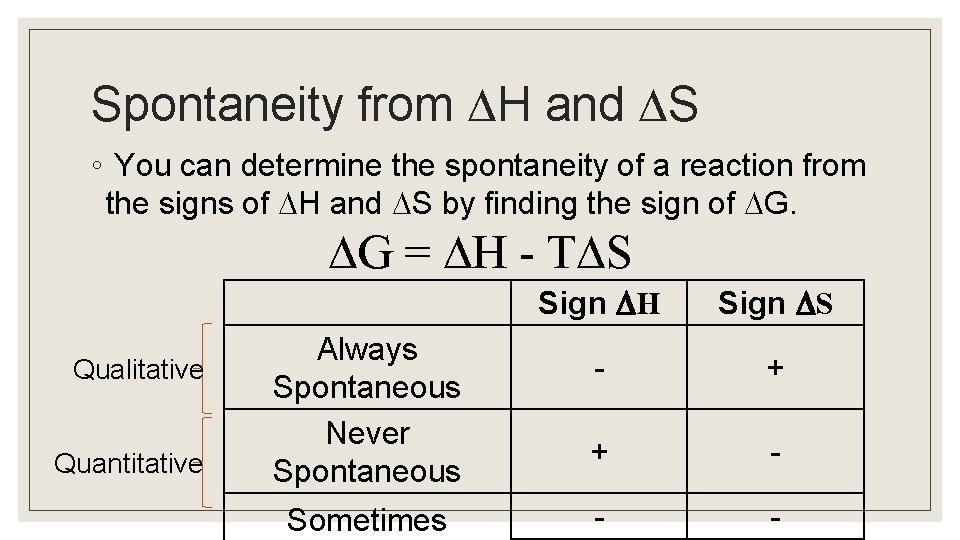
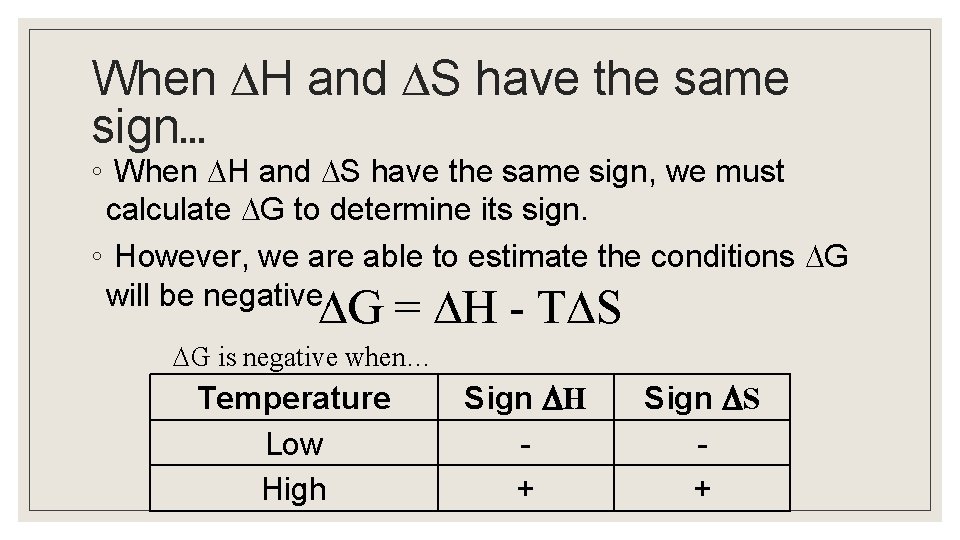
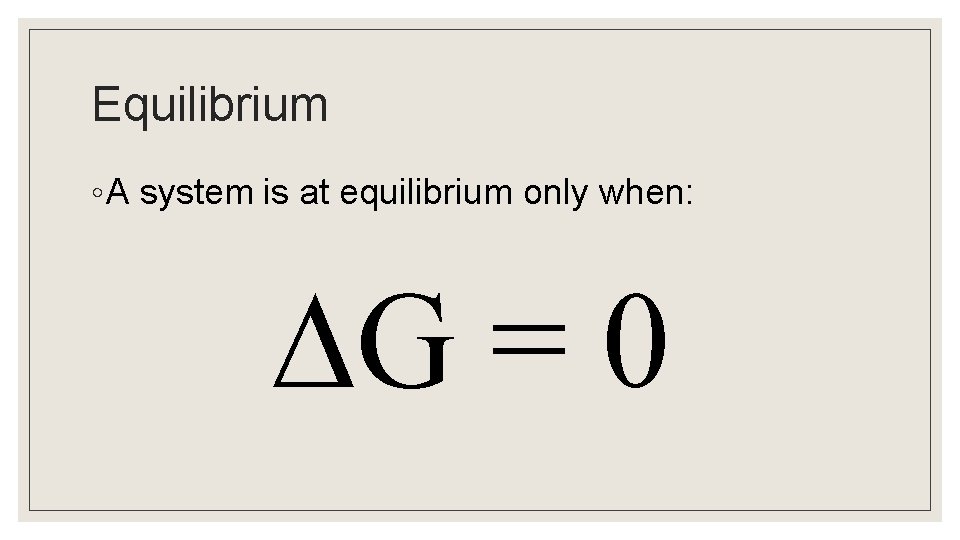
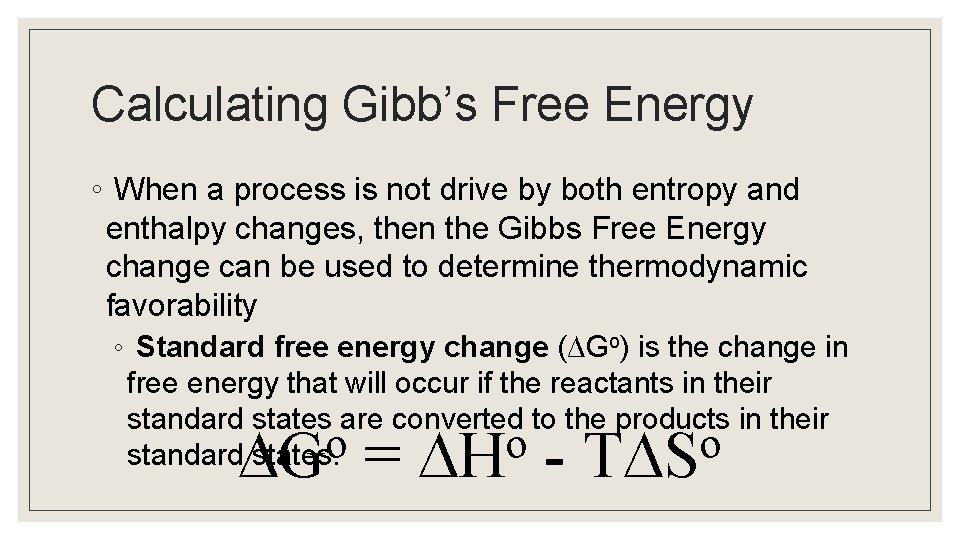
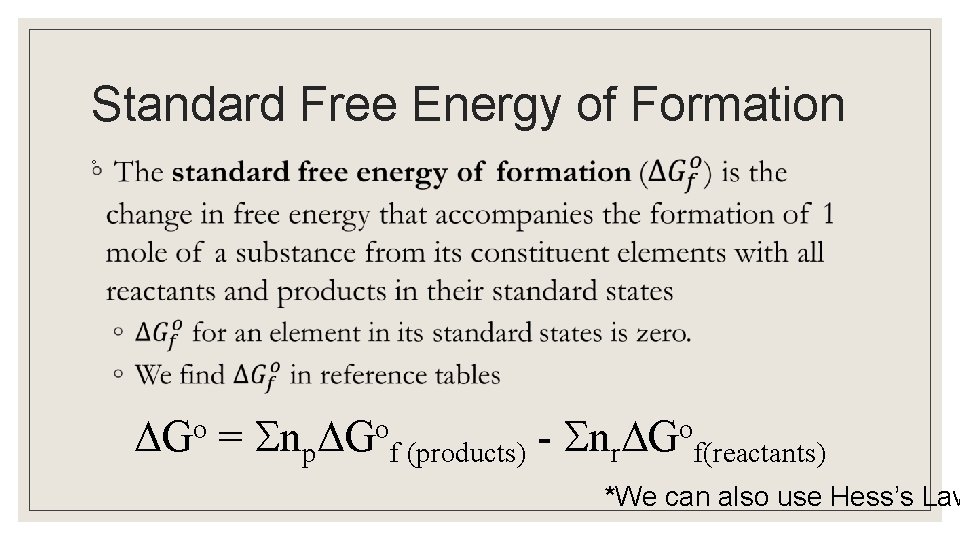
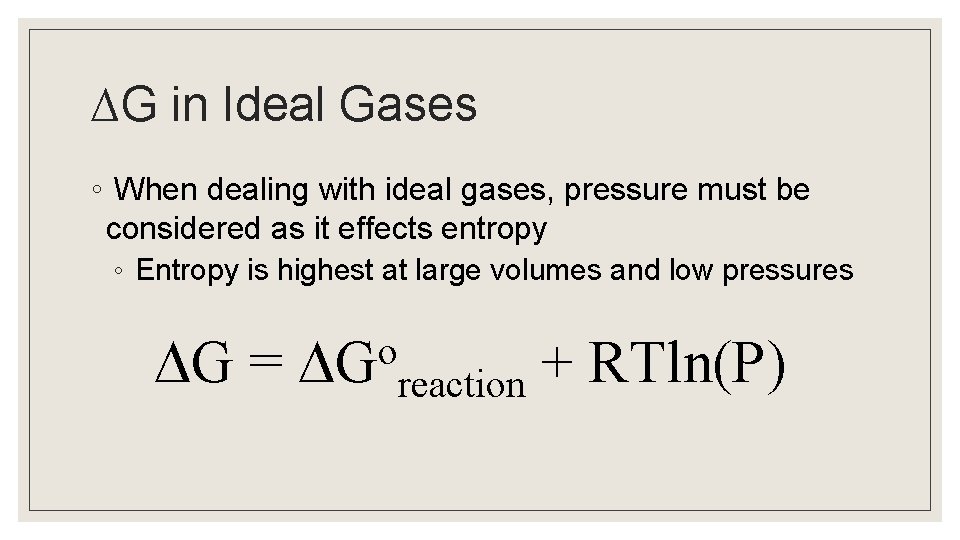
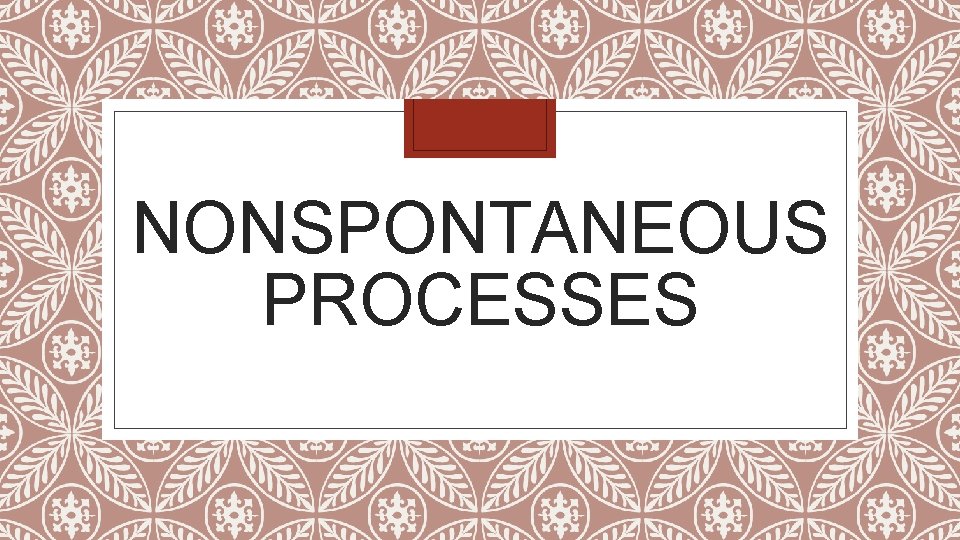
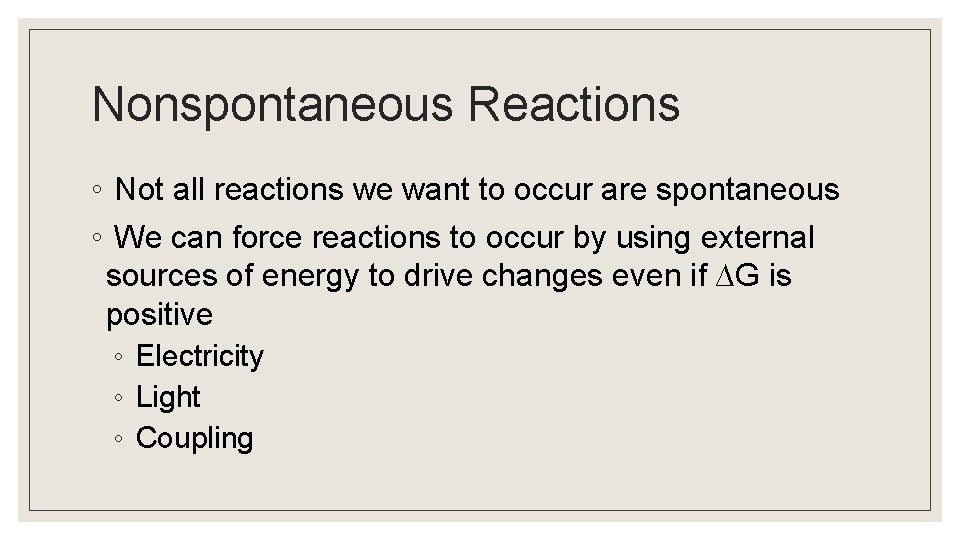
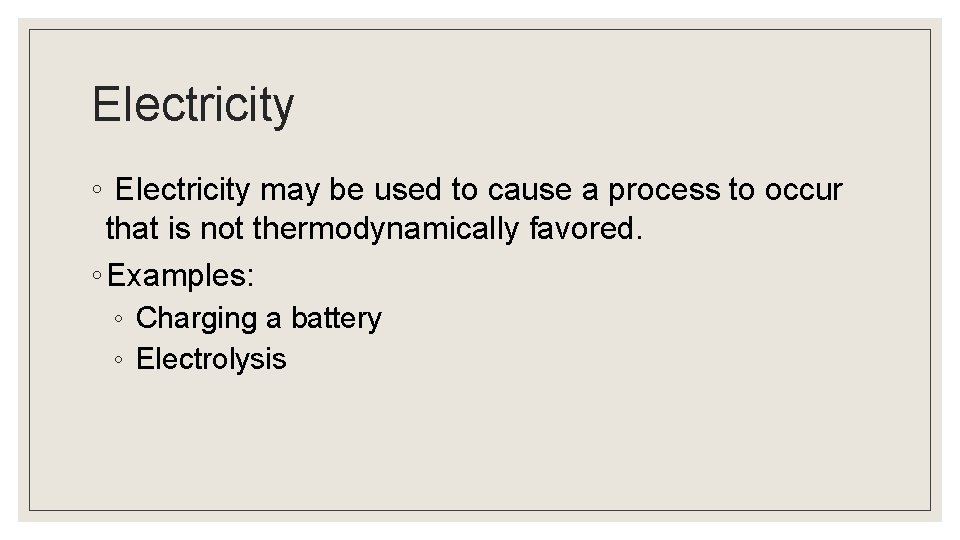
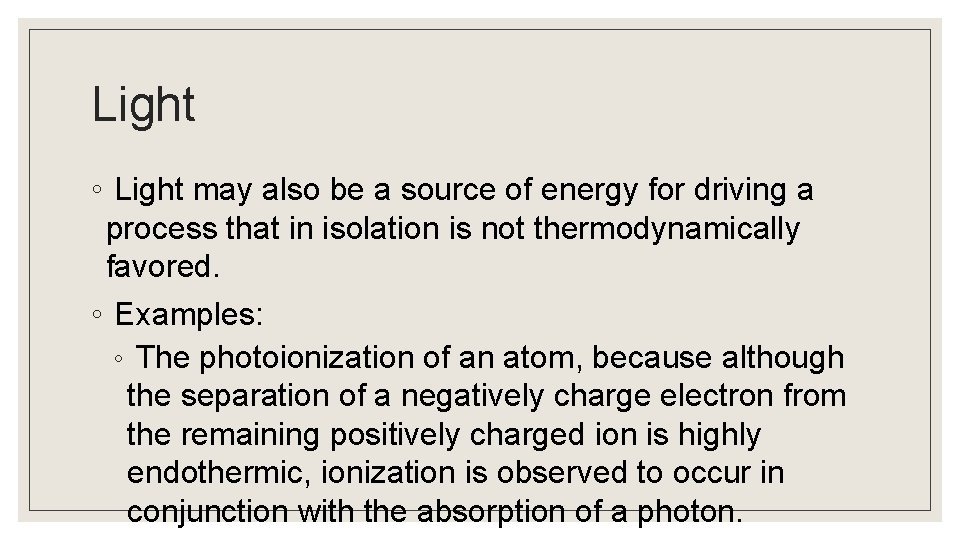
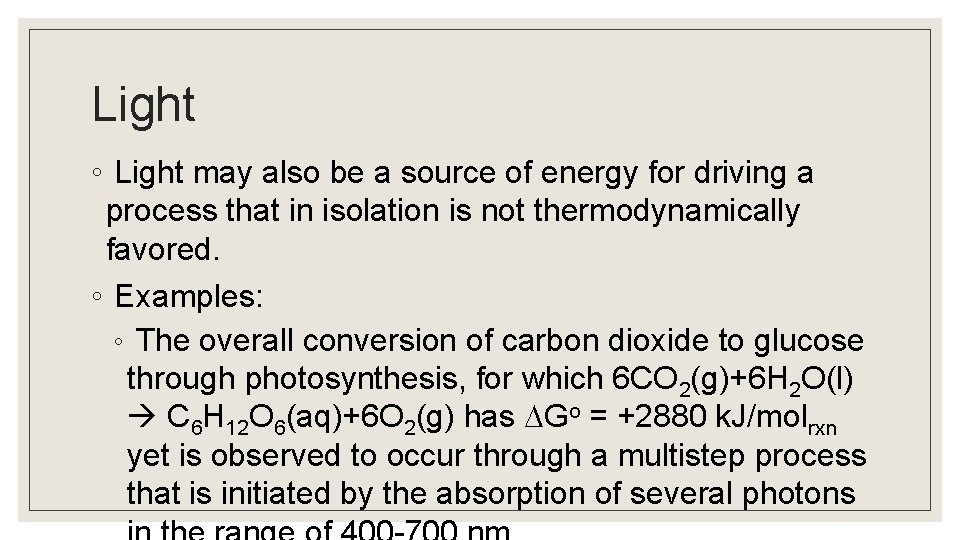
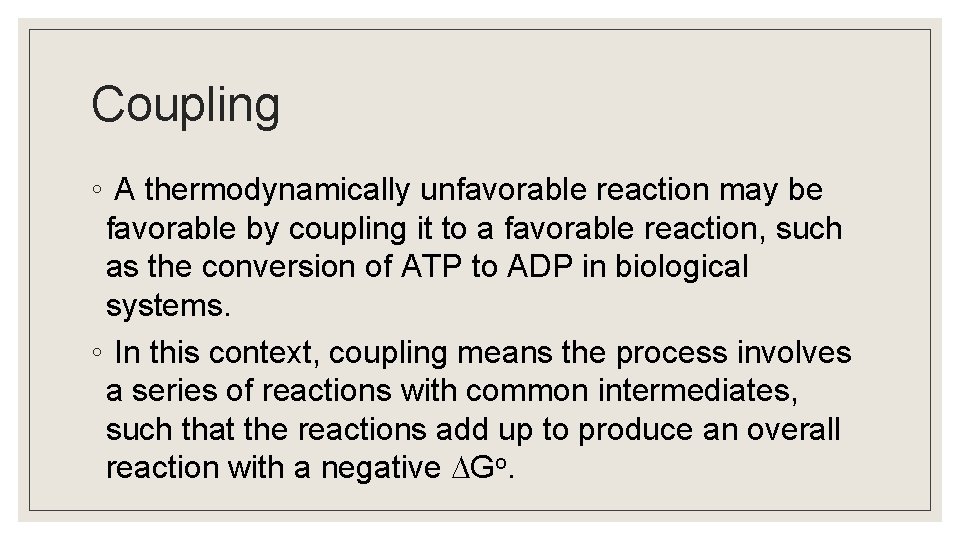
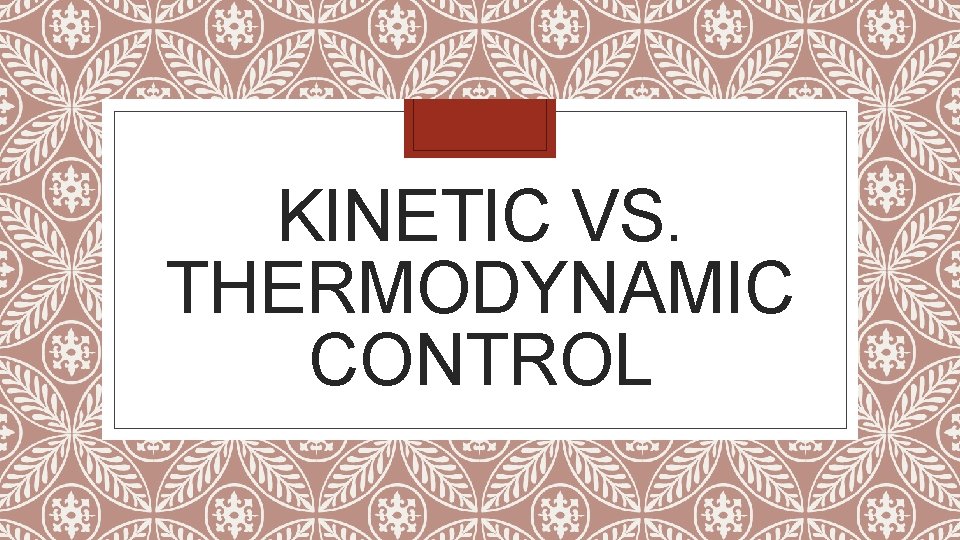
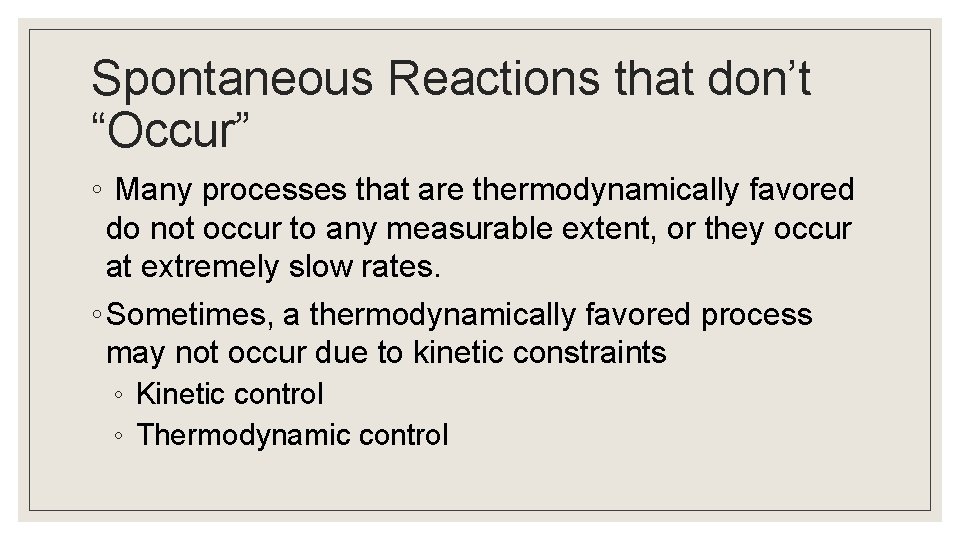
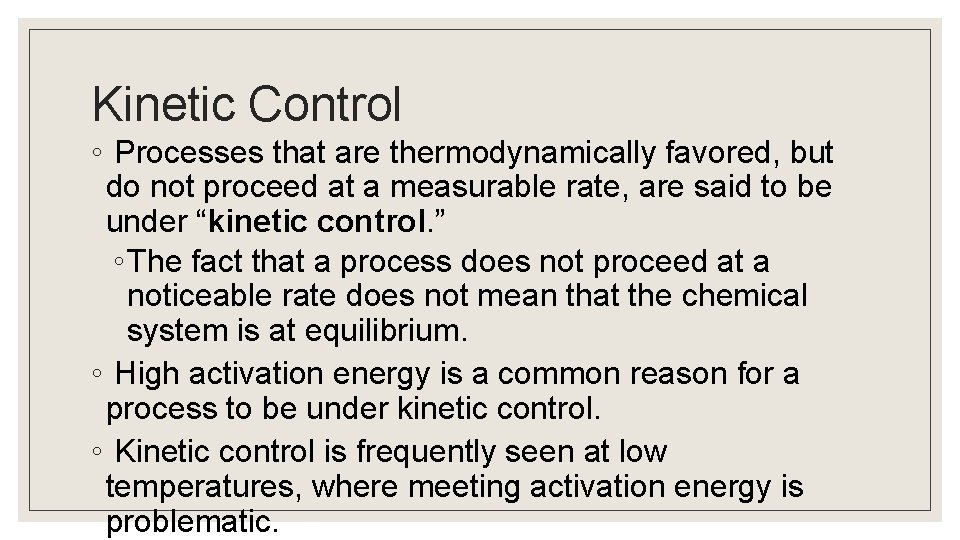
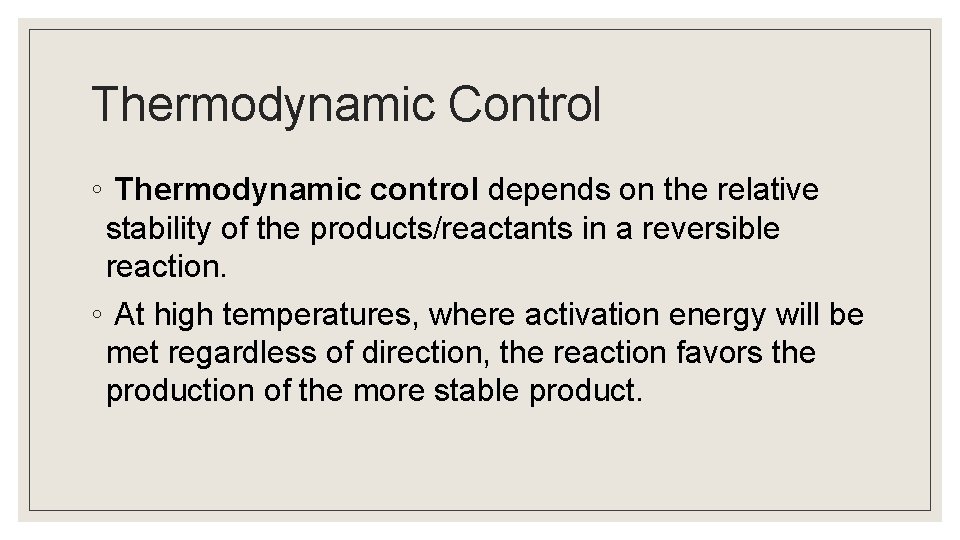
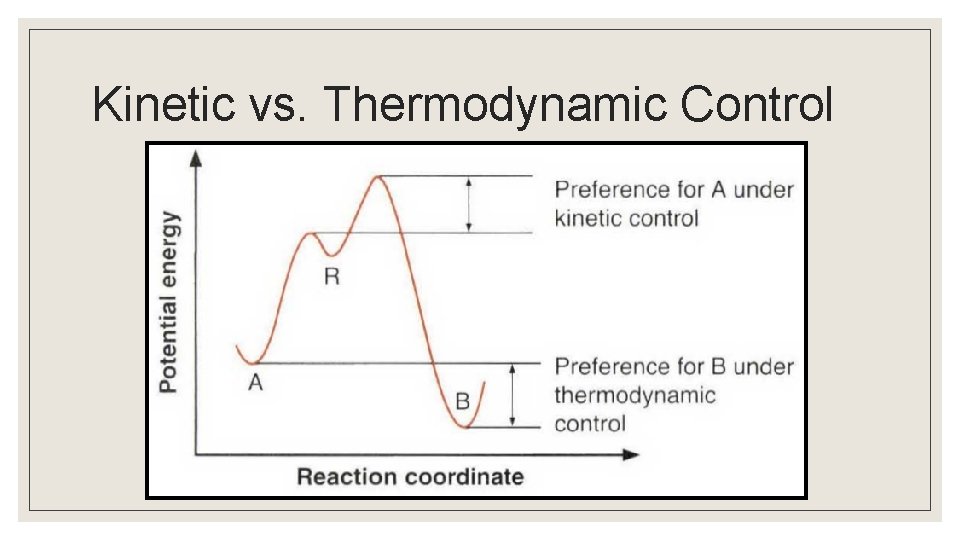
- Slides: 97
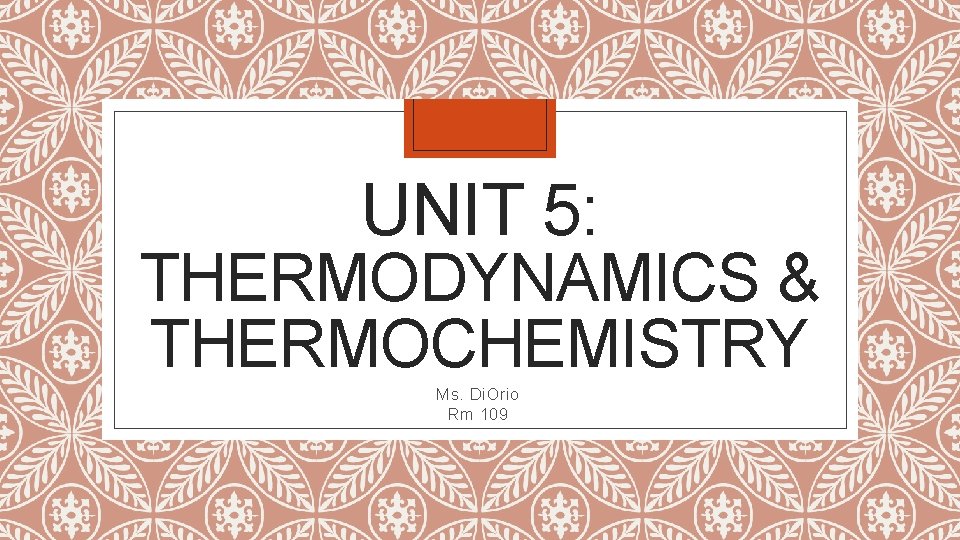
UNIT 5: THERMODYNAMICS & THERMOCHEMISTRY Ms. Di. Orio Rm 109
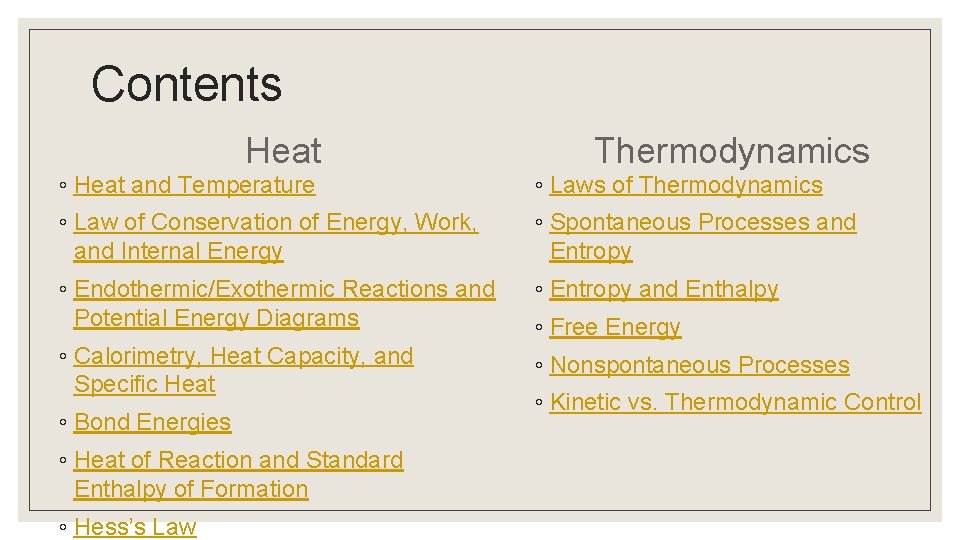
Contents Heat Thermodynamics ◦ Heat and Temperature ◦ Laws of Thermodynamics ◦ Law of Conservation of Energy, Work, and Internal Energy ◦ Spontaneous Processes and Entropy ◦ Endothermic/Exothermic Reactions and Potential Energy Diagrams ◦ Entropy and Enthalpy ◦ Calorimetry, Heat Capacity, and Specific Heat ◦ Nonspontaneous Processes ◦ Bond Energies ◦ Heat of Reaction and Standard Enthalpy of Formation ◦ Hess’s Law ◦ Free Energy ◦ Kinetic vs. Thermodynamic Control
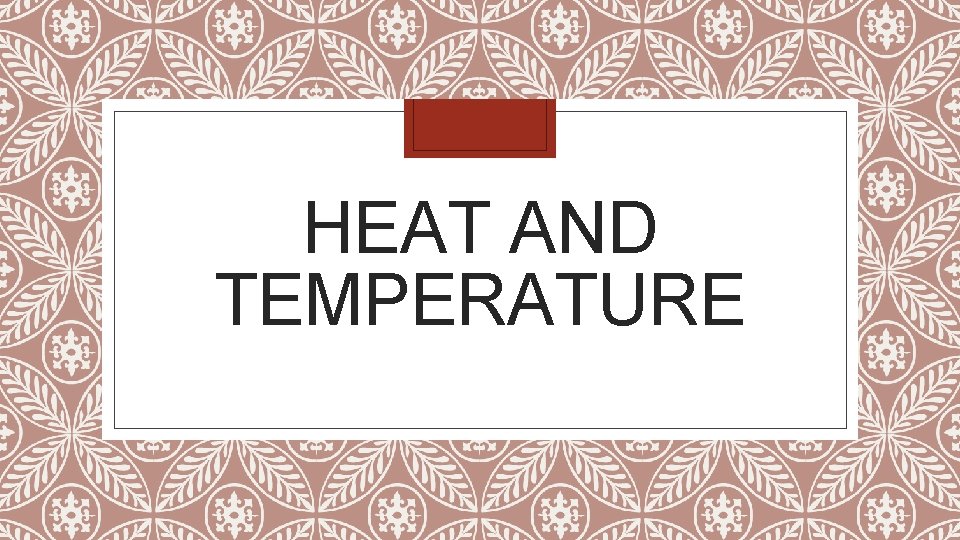
HEAT AND TEMPERATURE
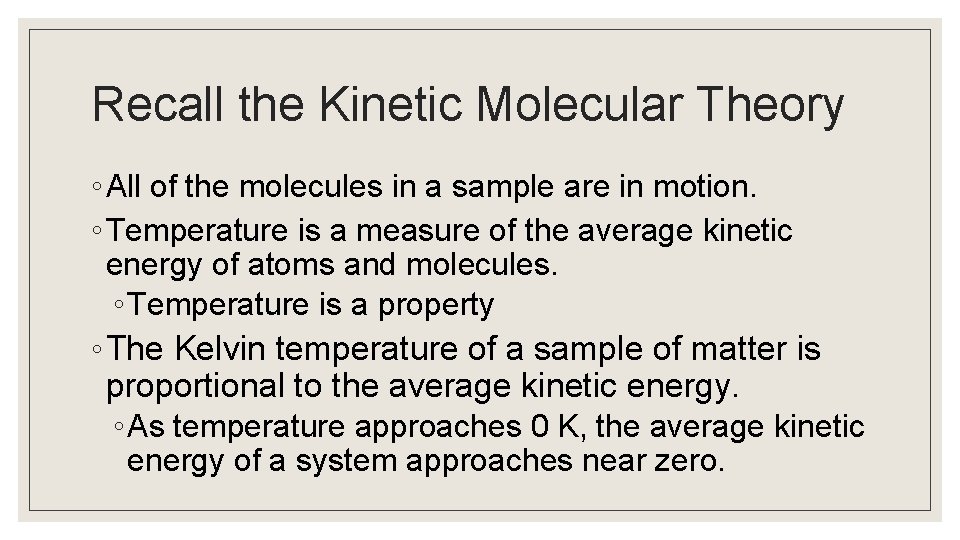
Recall the Kinetic Molecular Theory ◦ All of the molecules in a sample are in motion. ◦ Temperature is a measure of the average kinetic energy of atoms and molecules. ◦ Temperature is a property ◦ The Kelvin temperature of a sample of matter is proportional to the average kinetic energy. ◦ As temperature approaches 0 K, the average kinetic energy of a system approaches near zero.
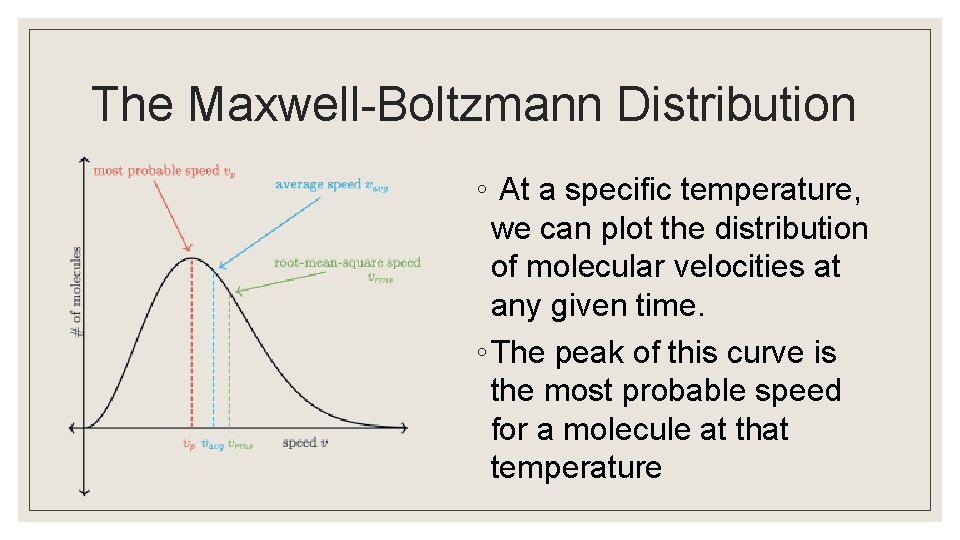
The Maxwell-Boltzmann Distribution ◦ At a specific temperature, we can plot the distribution of molecular velocities at any given time. ◦ The peak of this curve is the most probable speed for a molecule at that temperature
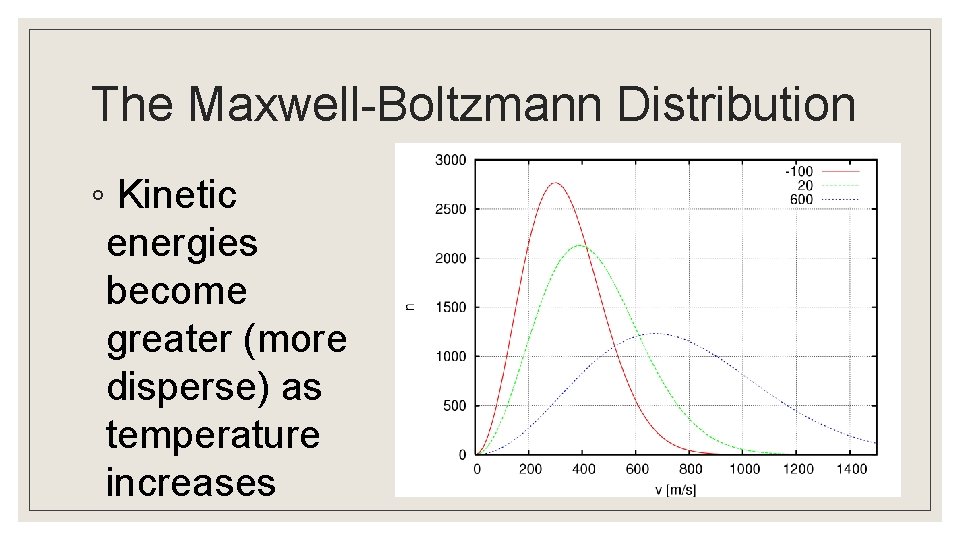
The Maxwell-Boltzmann Distribution ◦ Kinetic energies become greater (more disperse) as temperature increases
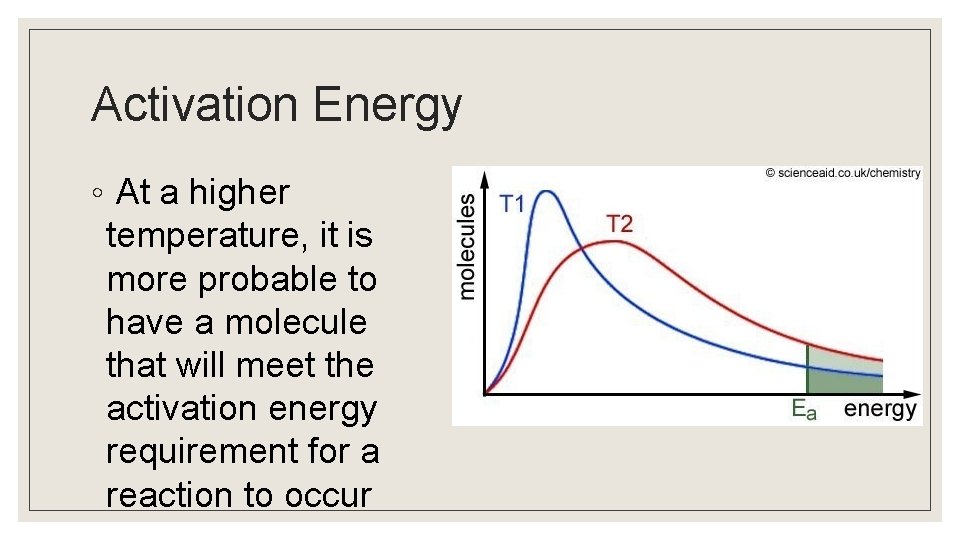
Activation Energy ◦ At a higher temperature, it is more probable to have a molecule that will meet the activation energy requirement for a reaction to occur
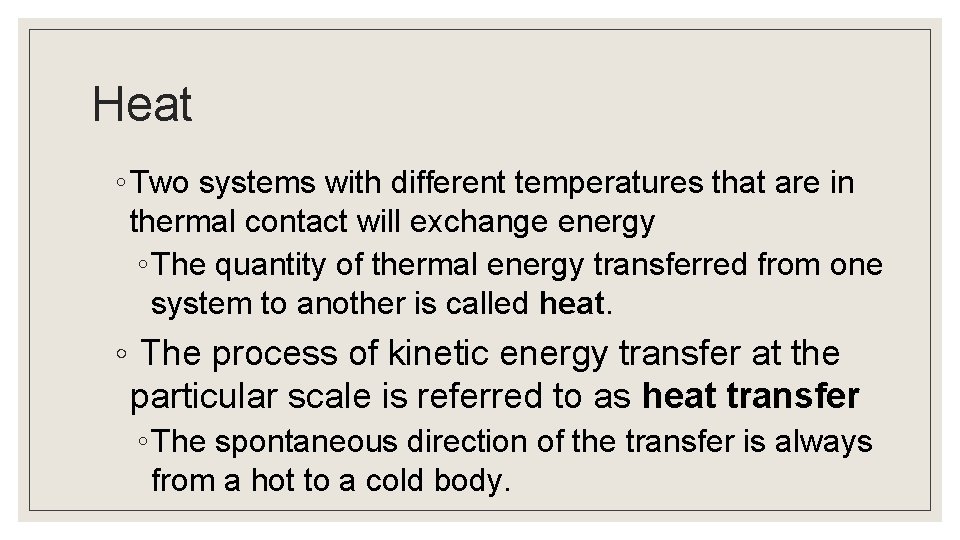
Heat ◦ Two systems with different temperatures that are in thermal contact will exchange energy ◦ The quantity of thermal energy transferred from one system to another is called heat. ◦ The process of kinetic energy transfer at the particular scale is referred to as heat transfer ◦ The spontaneous direction of the transfer is always from a hot to a cold body.
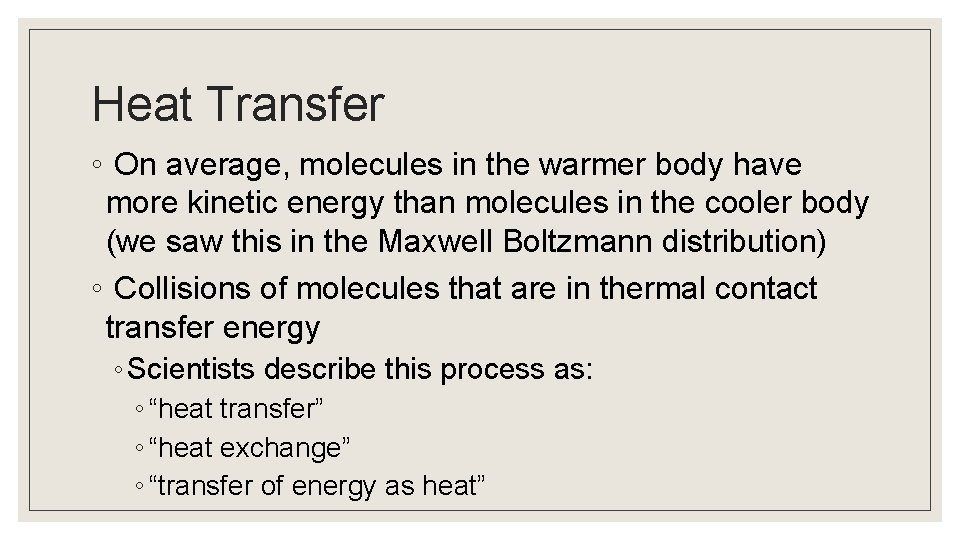
Heat Transfer ◦ On average, molecules in the warmer body have more kinetic energy than molecules in the cooler body (we saw this in the Maxwell Boltzmann distribution) ◦ Collisions of molecules that are in thermal contact transfer energy ◦ Scientists describe this process as: ◦ “heat transfer” ◦ “heat exchange” ◦ “transfer of energy as heat”
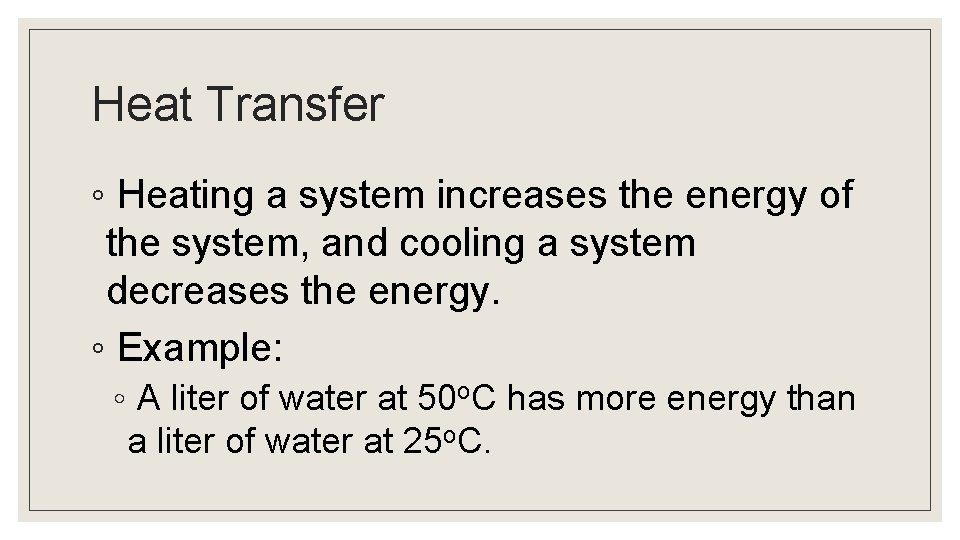
Heat Transfer ◦ Heating a system increases the energy of the system, and cooling a system decreases the energy. ◦ Example: ◦ A liter of water at 50 o. C has more energy than a liter of water at 25 o. C.
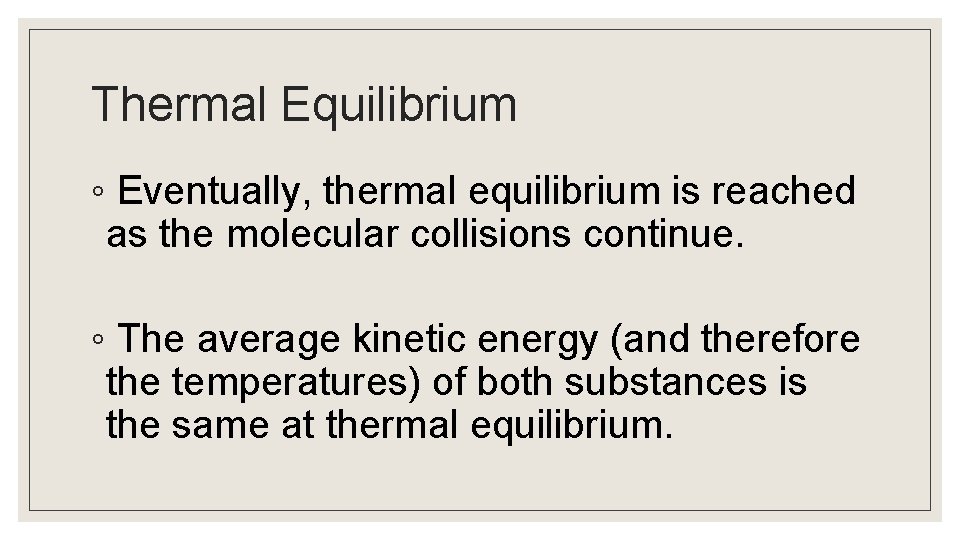
Thermal Equilibrium ◦ Eventually, thermal equilibrium is reached as the molecular collisions continue. ◦ The average kinetic energy (and therefore the temperatures) of both substances is the same at thermal equilibrium.
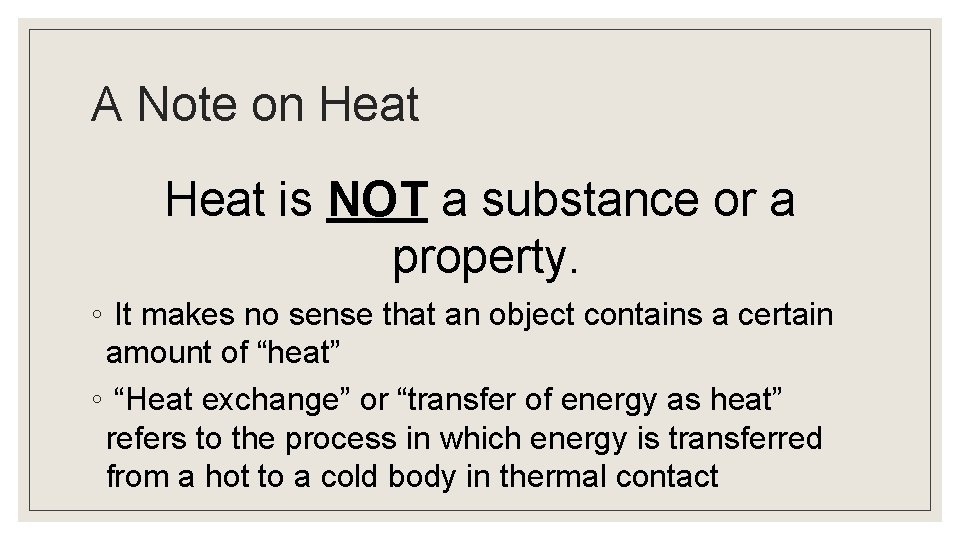
A Note on Heat is NOT a substance or a property. ◦ It makes no sense that an object contains a certain amount of “heat” ◦ “Heat exchange” or “transfer of energy as heat” refers to the process in which energy is transferred from a hot to a cold body in thermal contact
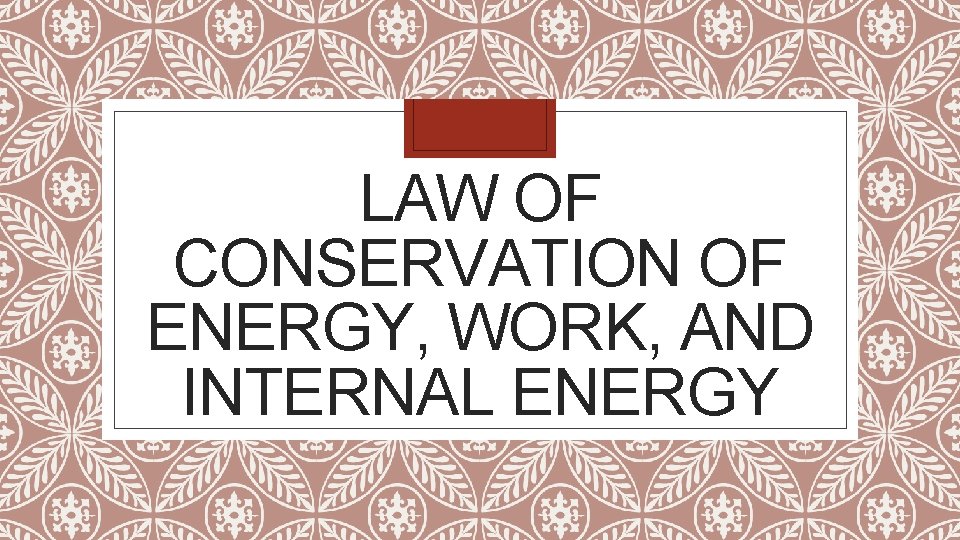
LAW OF CONSERVATION OF ENERGY, WORK, AND INTERNAL ENERGY
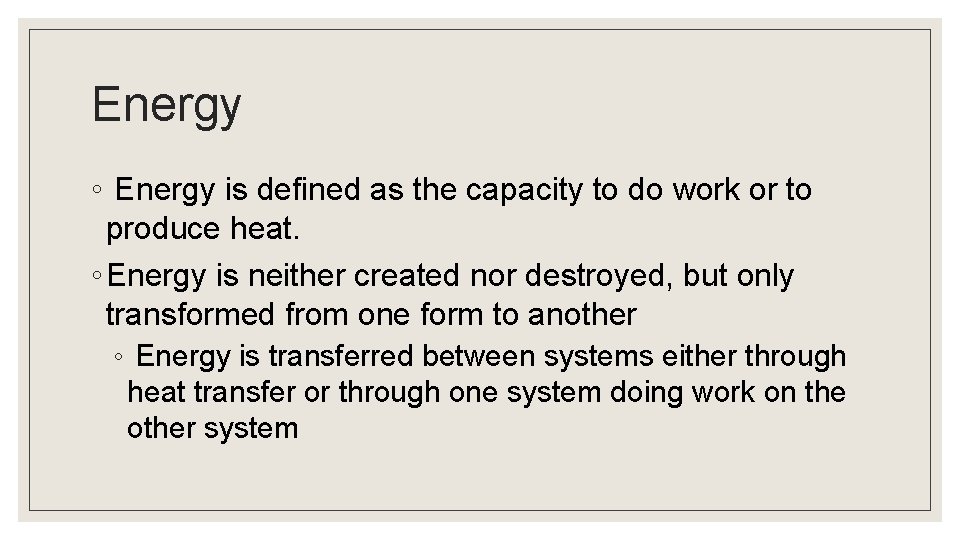
Energy ◦ Energy is defined as the capacity to do work or to produce heat. ◦ Energy is neither created nor destroyed, but only transformed from one form to another ◦ Energy is transferred between systems either through heat transfer or through one system doing work on the other system
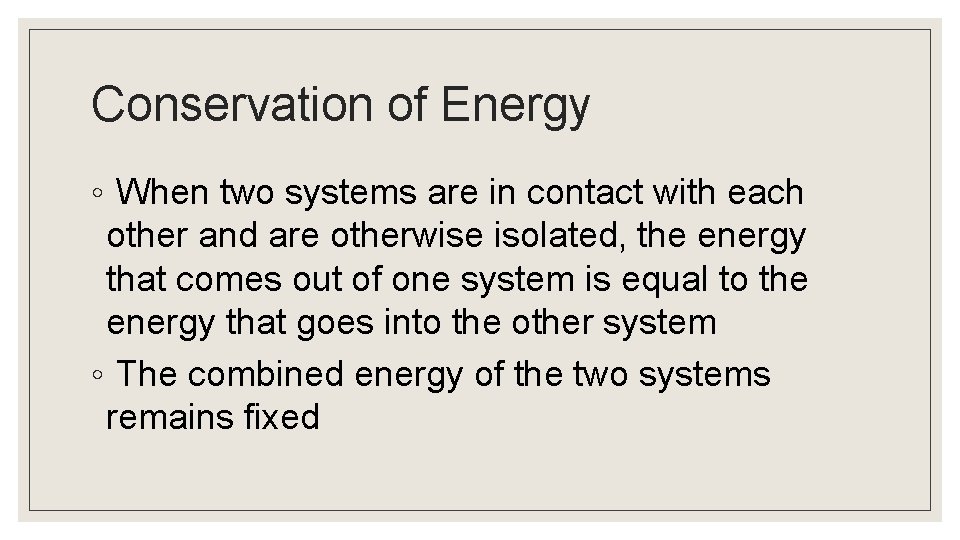
Conservation of Energy ◦ When two systems are in contact with each other and are otherwise isolated, the energy that comes out of one system is equal to the energy that goes into the other system ◦ The combined energy of the two systems remains fixed
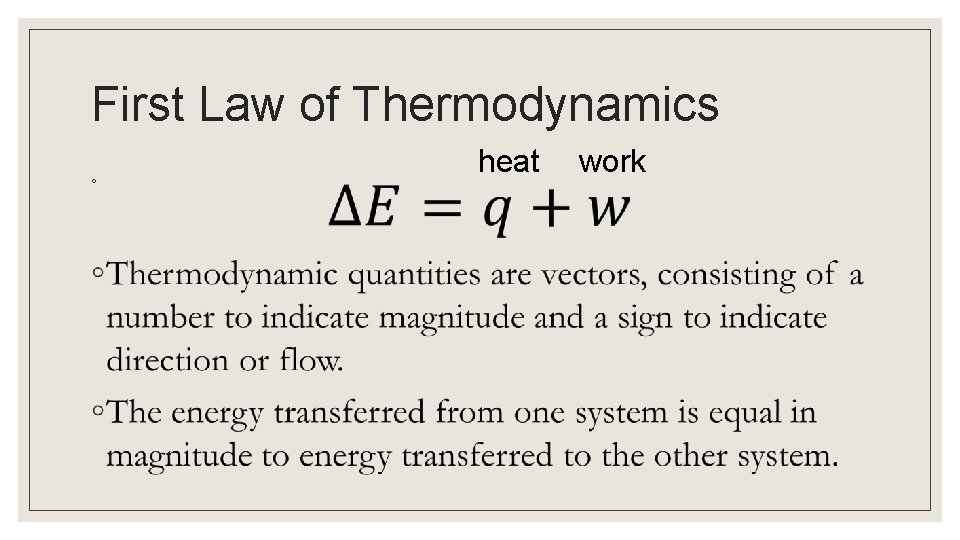
First Law of Thermodynamics ◦ heat work
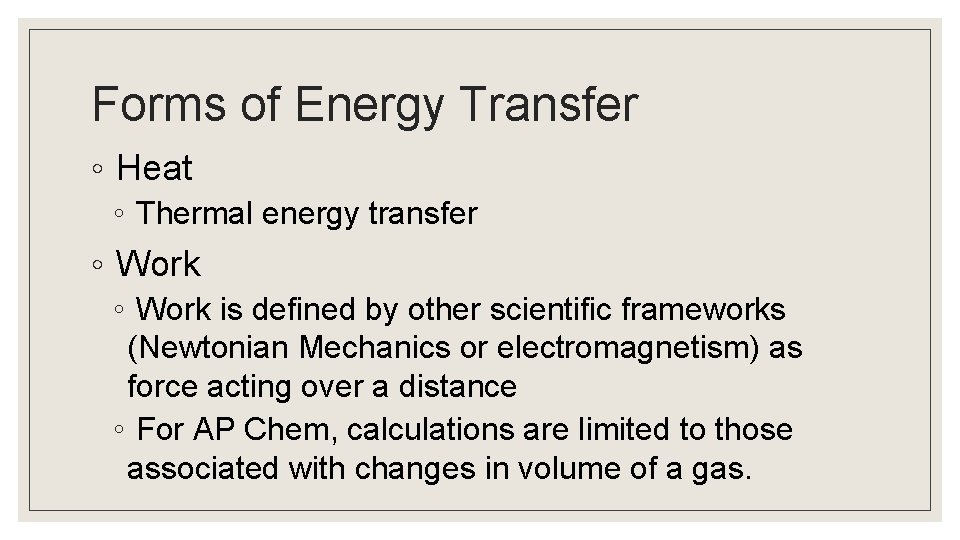
Forms of Energy Transfer ◦ Heat ◦ Thermal energy transfer ◦ Work is defined by other scientific frameworks (Newtonian Mechanics or electromagnetism) as force acting over a distance ◦ For AP Chem, calculations are limited to those associated with changes in volume of a gas.
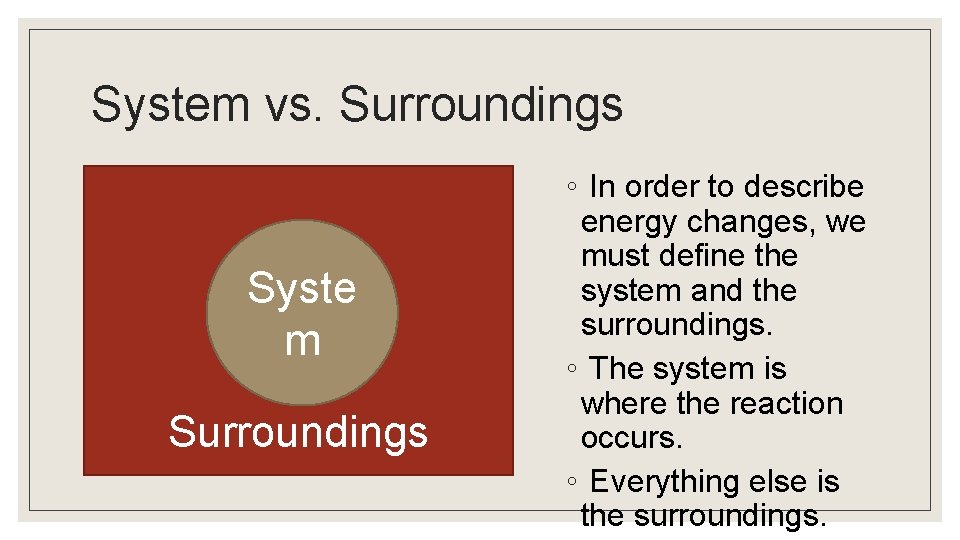
System vs. Surroundings Syste m Surroundings ◦ In order to describe energy changes, we must define the system and the surroundings. ◦ The system is where the reaction occurs. ◦ Everything else is the surroundings.
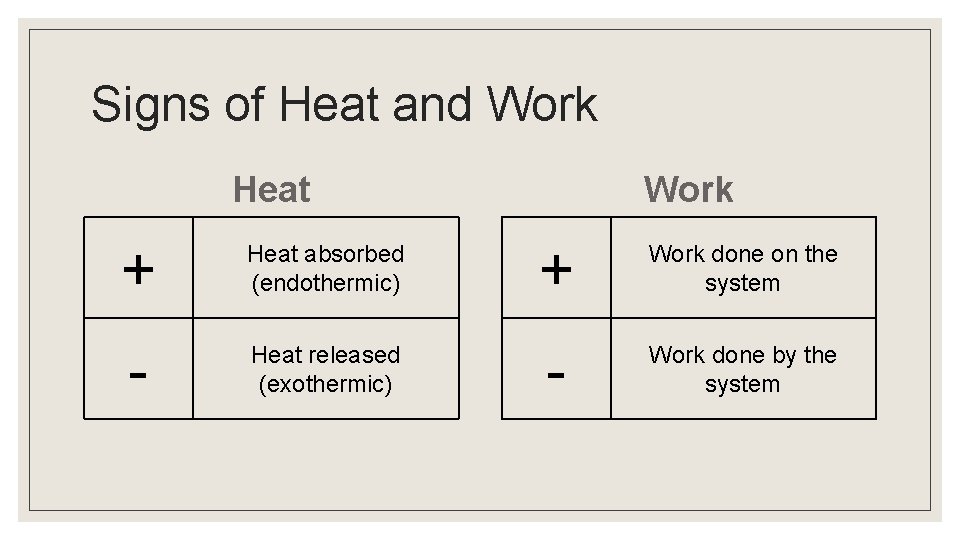
Signs of Heat and Work Heat Work + Heat absorbed (endothermic) + Work done on the system - Heat released (exothermic) - Work done by the system
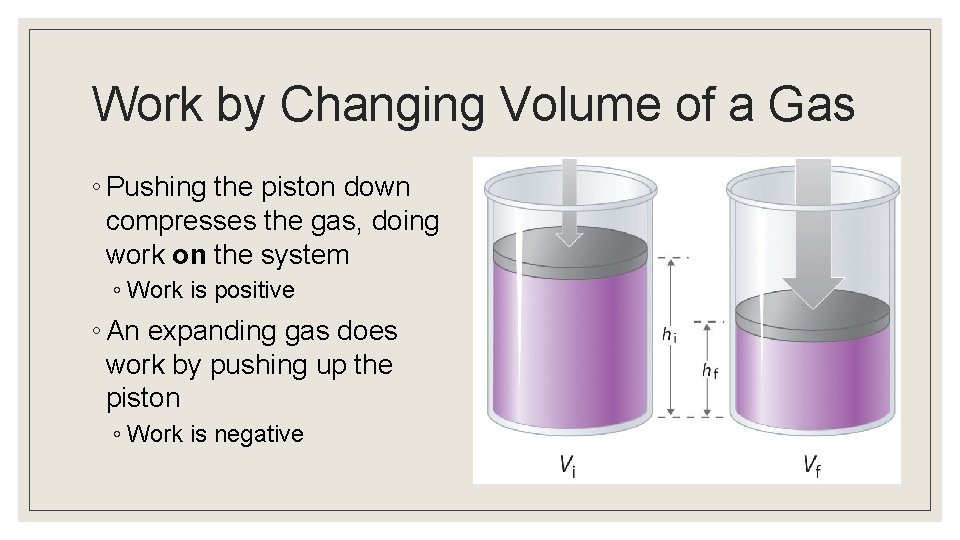
Work by Changing Volume of a Gas ◦ Pushing the piston down compresses the gas, doing work on the system ◦ Work is positive ◦ An expanding gas does work by pushing up the piston ◦ Work is negative
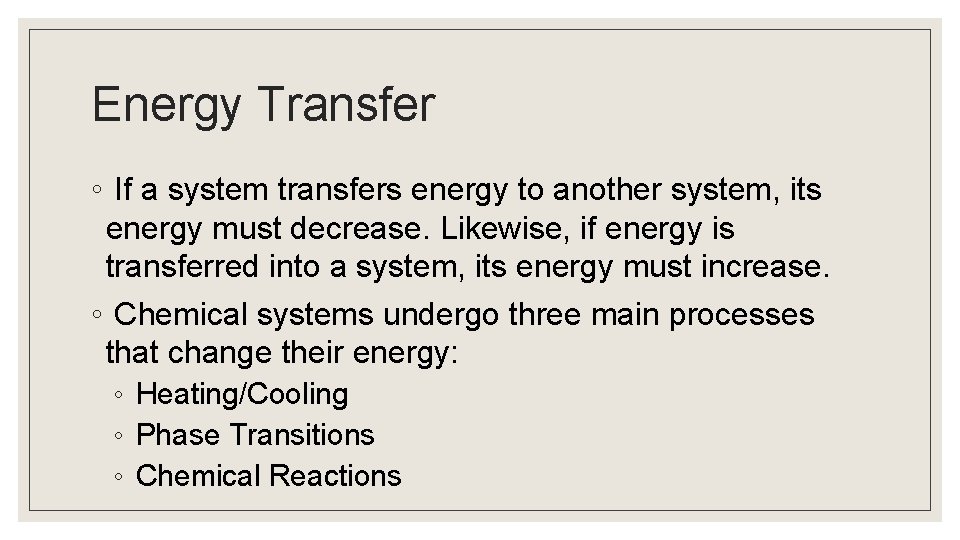
Energy Transfer ◦ If a system transfers energy to another system, its energy must decrease. Likewise, if energy is transferred into a system, its energy must increase. ◦ Chemical systems undergo three main processes that change their energy: ◦ Heating/Cooling ◦ Phase Transitions ◦ Chemical Reactions
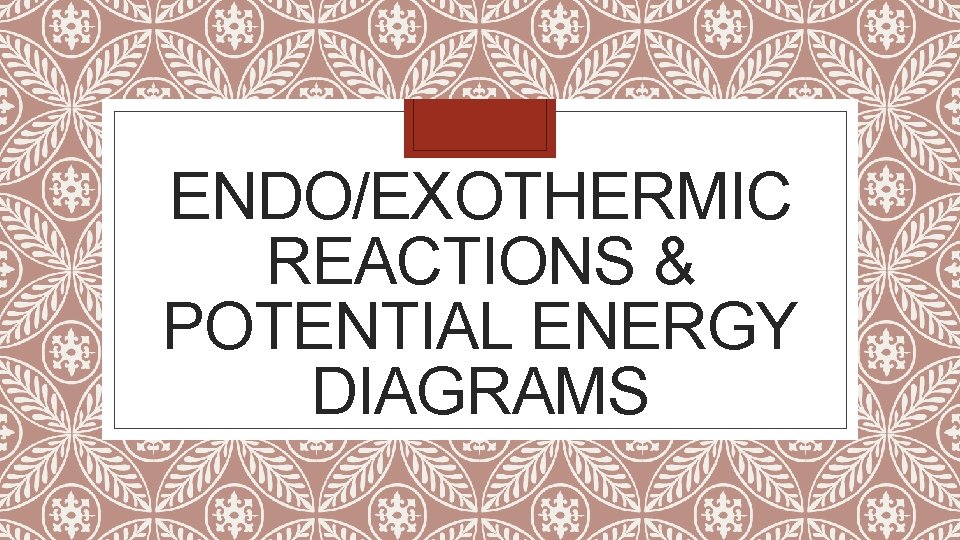
ENDO/EXOTHERMIC REACTIONS & POTENTIAL ENERGY DIAGRAMS
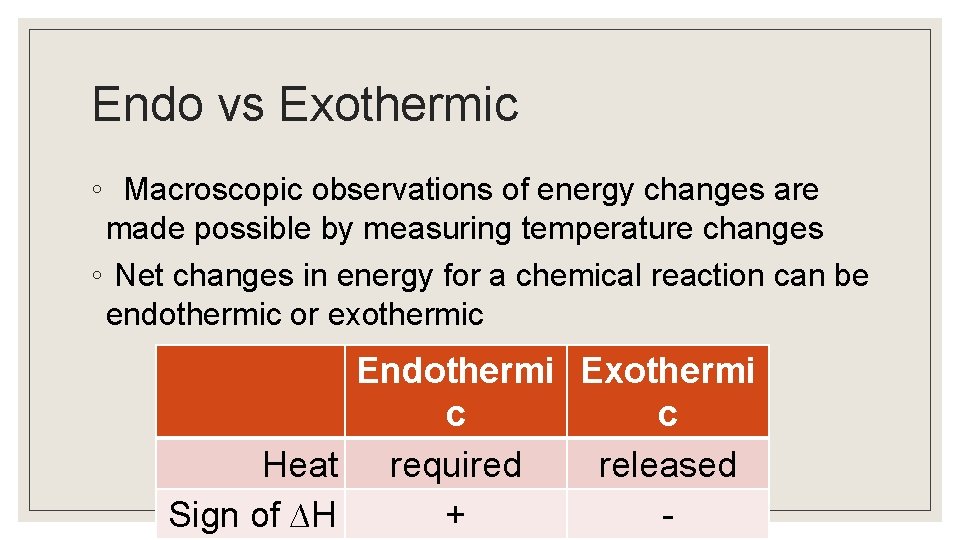
Endo vs Exothermic ◦ Macroscopic observations of energy changes are made possible by measuring temperature changes ◦ Net changes in energy for a chemical reaction can be endothermic or exothermic Endothermi Exothermi c c Heat required released Sign of ∆H + -
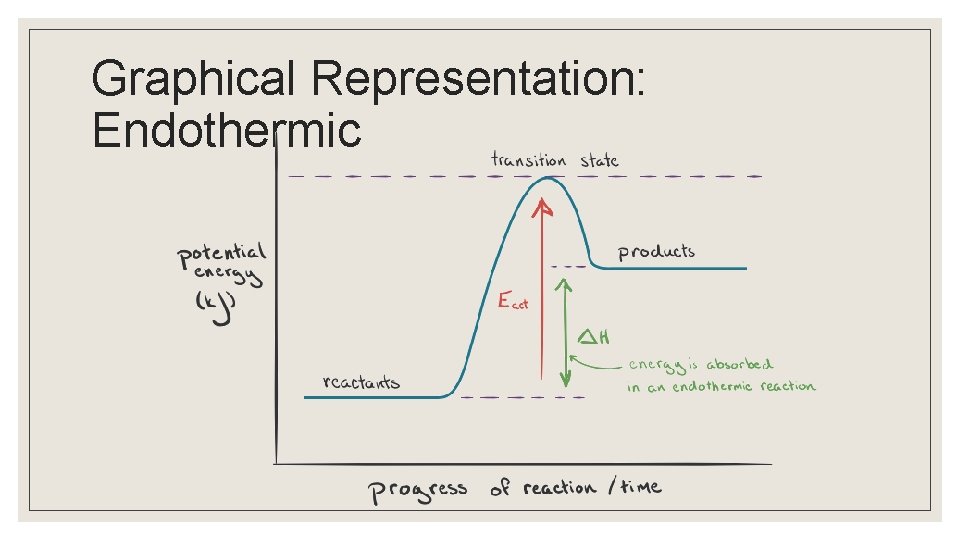
Graphical Representation: Endothermic
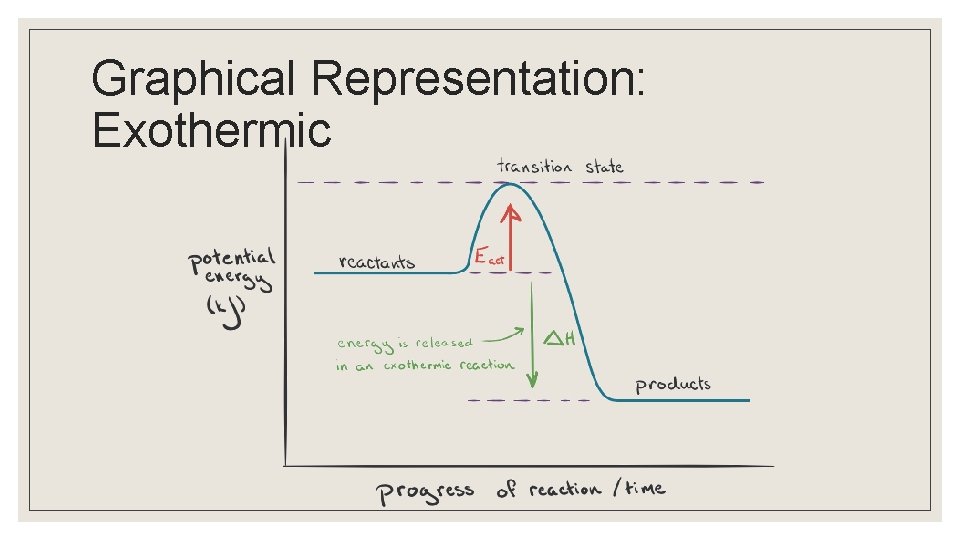
Graphical Representation: Exothermic
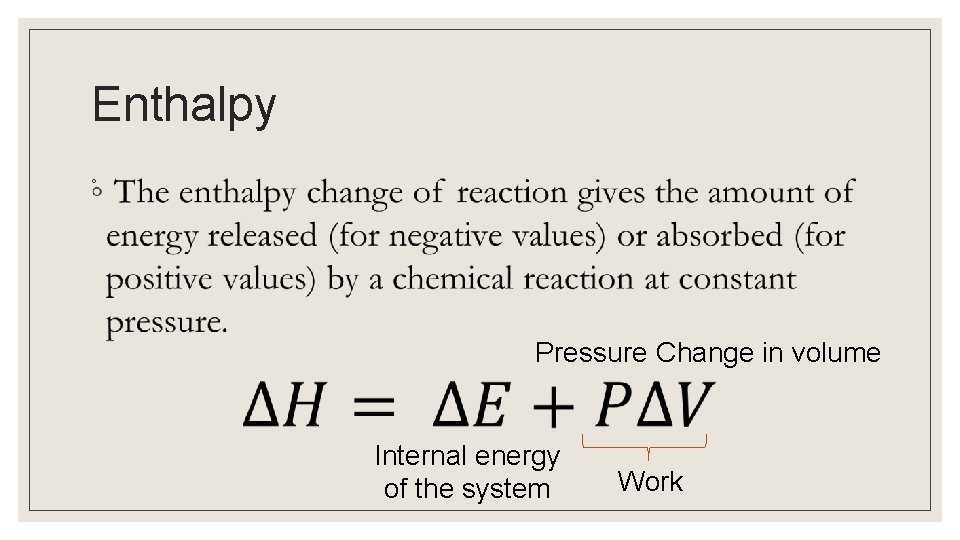
Enthalpy ◦ Pressure Change in volume Internal energy of the system Work
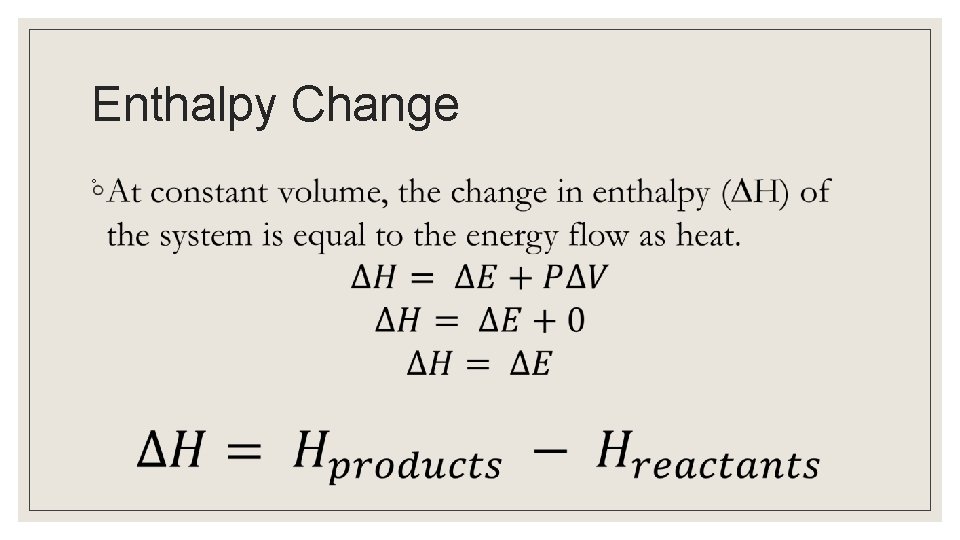
Enthalpy Change ◦
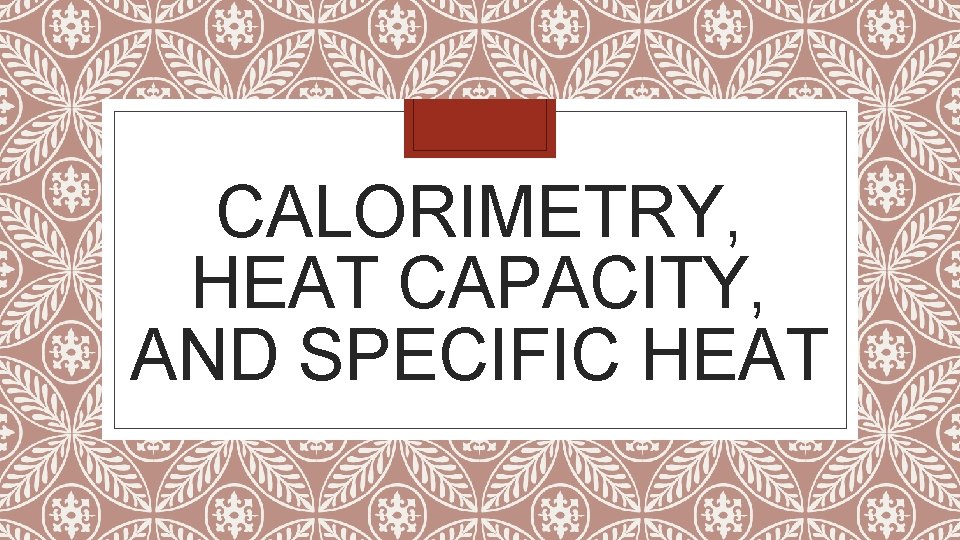
CALORIMETRY, HEAT CAPACITY, AND SPECIFIC HEAT
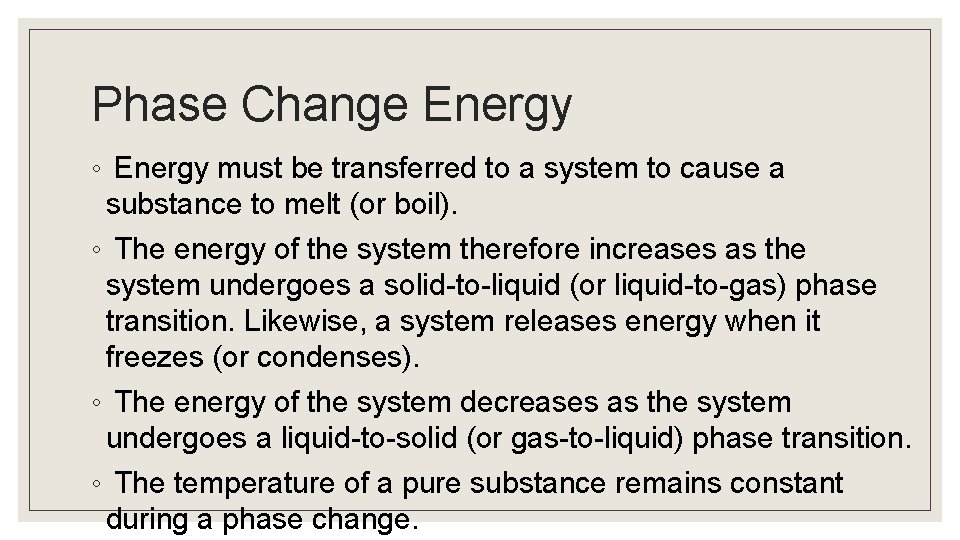
Phase Change Energy ◦ Energy must be transferred to a system to cause a substance to melt (or boil). ◦ The energy of the system therefore increases as the system undergoes a solid-to-liquid (or liquid-to-gas) phase transition. Likewise, a system releases energy when it freezes (or condenses). ◦ The energy of the system decreases as the system undergoes a liquid-to-solid (or gas-to-liquid) phase transition. ◦ The temperature of a pure substance remains constant during a phase change.
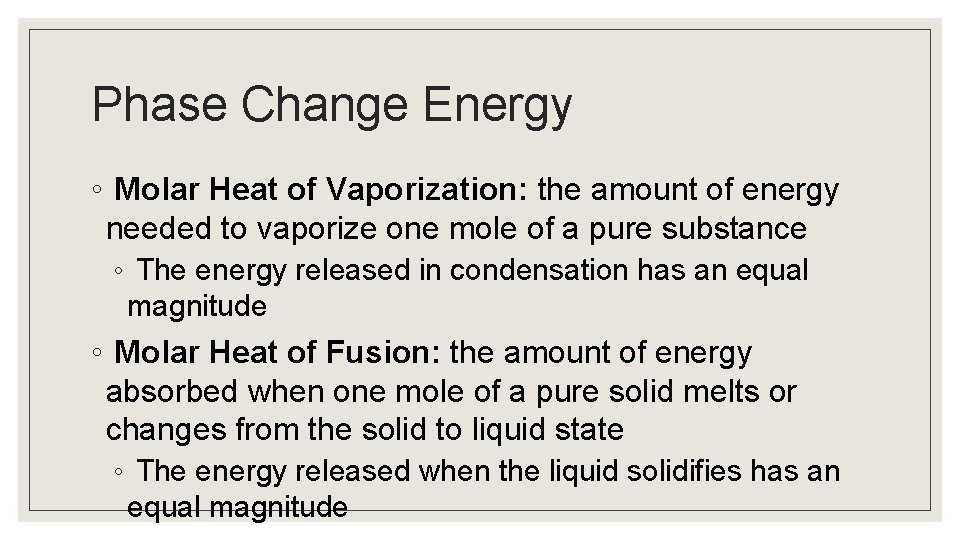
Phase Change Energy ◦ Molar Heat of Vaporization: the amount of energy needed to vaporize one mole of a pure substance ◦ The energy released in condensation has an equal magnitude ◦ Molar Heat of Fusion: the amount of energy absorbed when one mole of a pure solid melts or changes from the solid to liquid state ◦ The energy released when the liquid solidifies has an equal magnitude
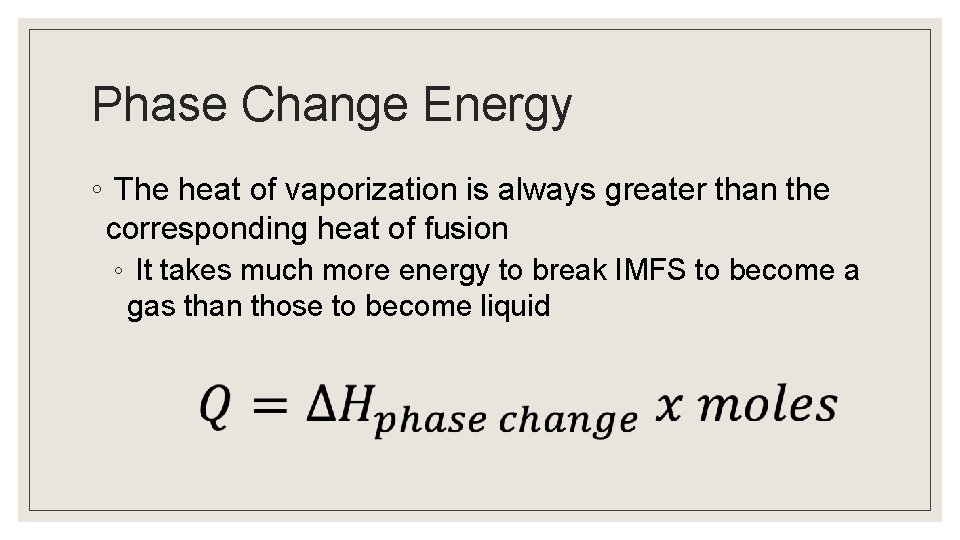
Phase Change Energy ◦ The heat of vaporization is always greater than the corresponding heat of fusion ◦ It takes much more energy to break IMFS to become a gas than those to become liquid
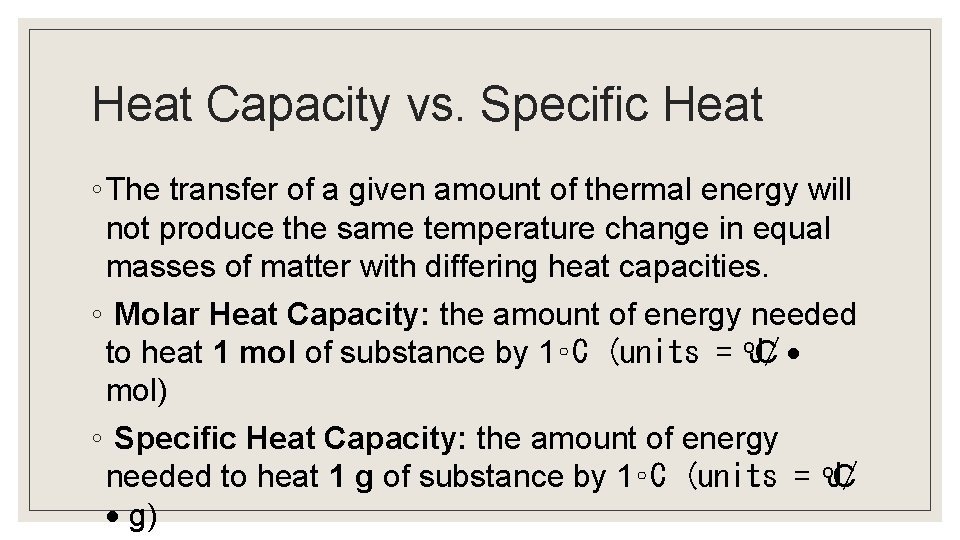
Heat Capacity vs. Specific Heat ◦ The transfer of a given amount of thermal energy will not produce the same temperature change in equal masses of matter with differing heat capacities. ◦ Molar Heat Capacity: the amount of energy needed to heat 1 mol of substance by 1∘C (units = o. J/ C mol) ◦ Specific Heat Capacity: the amount of energy needed to heat 1 g of substance by 1∘C (units = o. J/ C g)
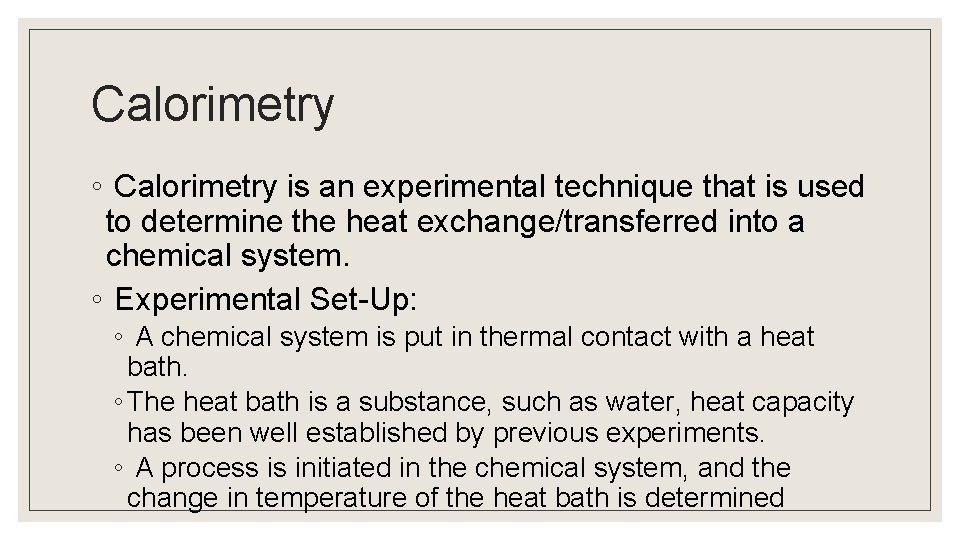
Calorimetry ◦ Calorimetry is an experimental technique that is used to determine the heat exchange/transferred into a chemical system. ◦ Experimental Set-Up: ◦ A chemical system is put in thermal contact with a heat bath. ◦ The heat bath is a substance, such as water, heat capacity has been well established by previous experiments. ◦ A process is initiated in the chemical system, and the change in temperature of the heat bath is determined
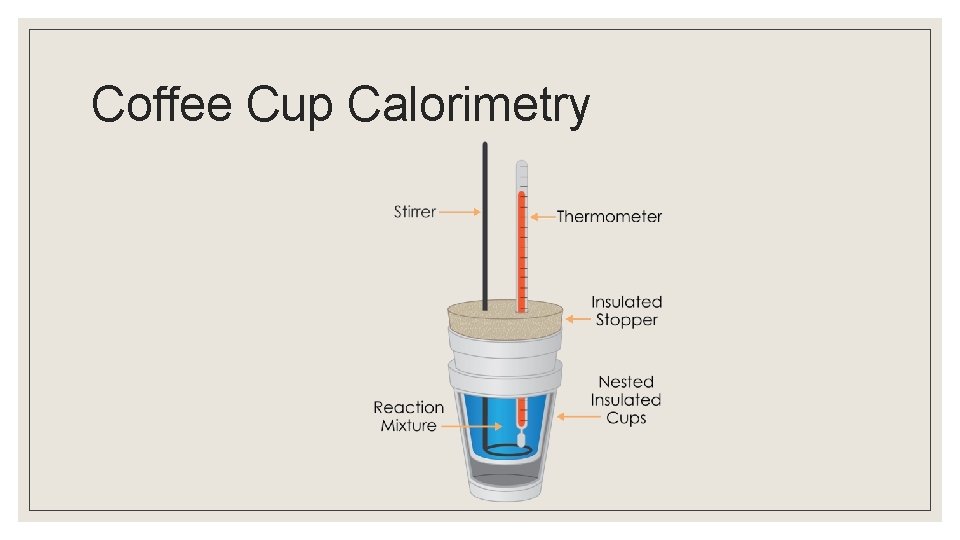
Coffee Cup Calorimetry
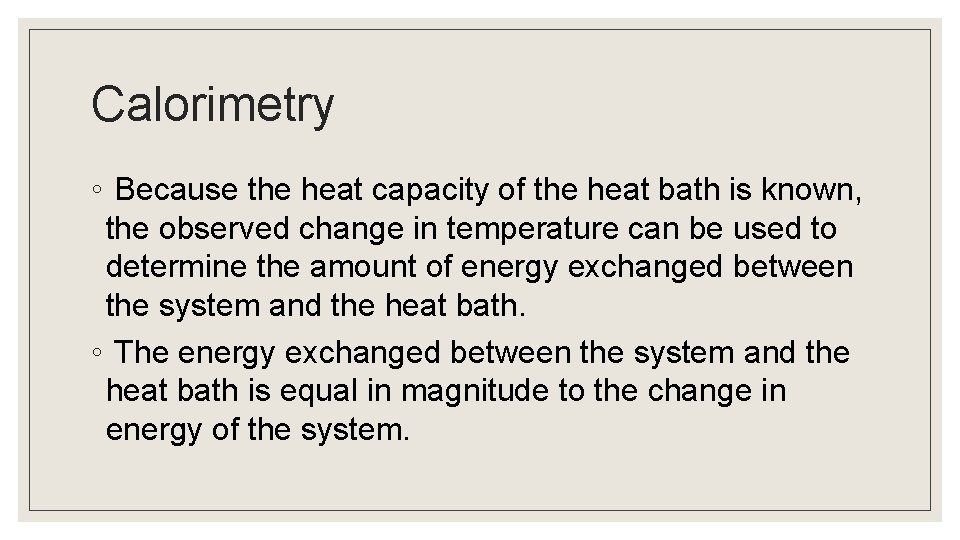
Calorimetry ◦ Because the heat capacity of the heat bath is known, the observed change in temperature can be used to determine the amount of energy exchanged between the system and the heat bath. ◦ The energy exchanged between the system and the heat bath is equal in magnitude to the change in energy of the system.
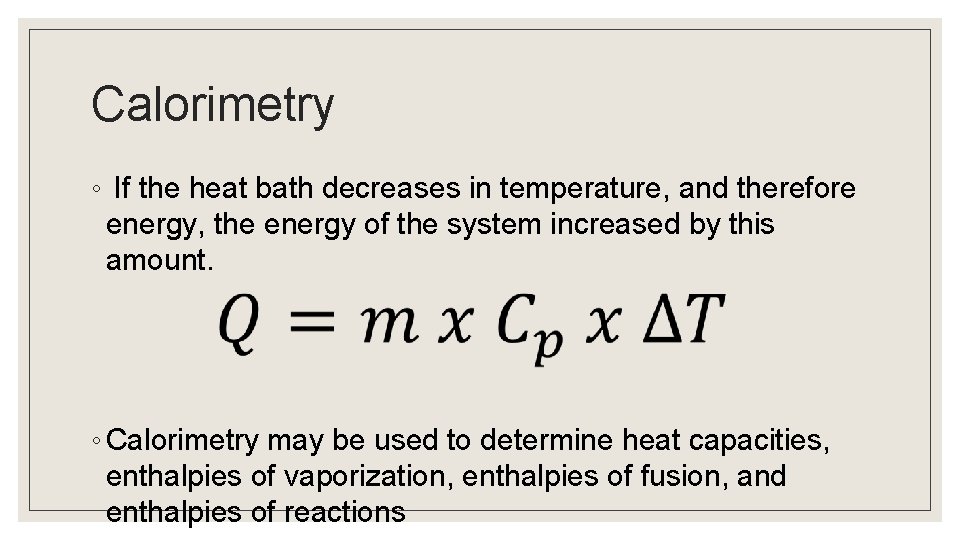
Calorimetry ◦ If the heat bath decreases in temperature, and therefore energy, the energy of the system increased by this amount. ◦ Calorimetry may be used to determine heat capacities, enthalpies of vaporization, enthalpies of fusion, and enthalpies of reactions
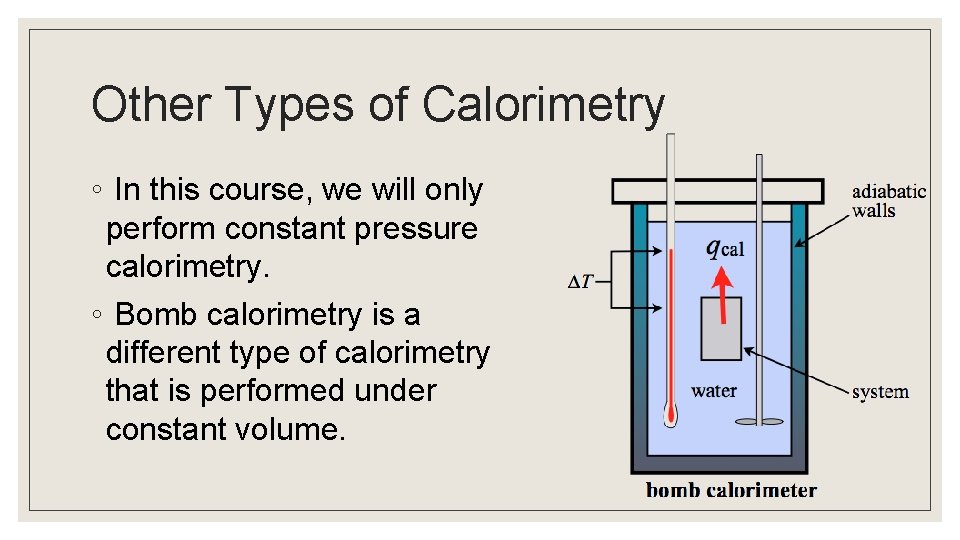
Other Types of Calorimetry ◦ In this course, we will only perform constant pressure calorimetry. ◦ Bomb calorimetry is a different type of calorimetry that is performed under constant volume.
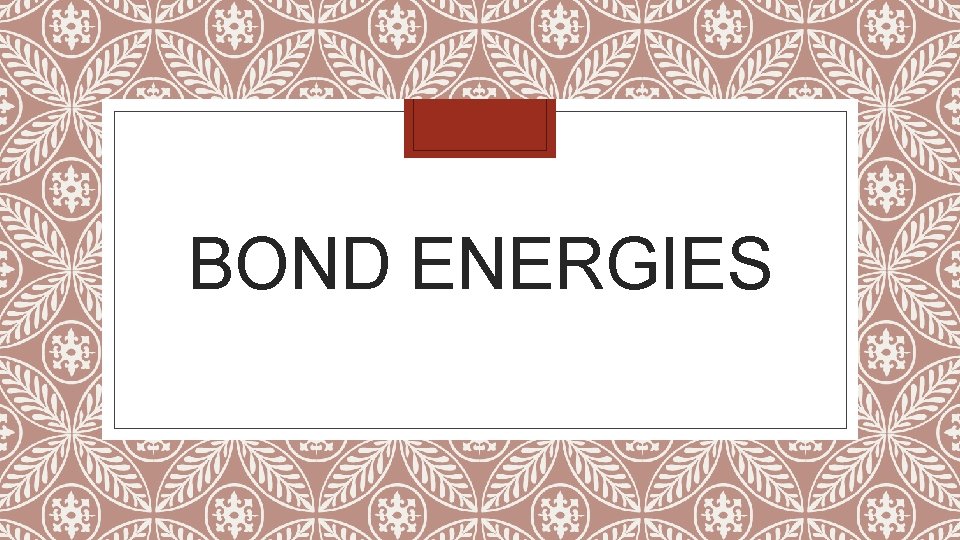
BOND ENERGIES
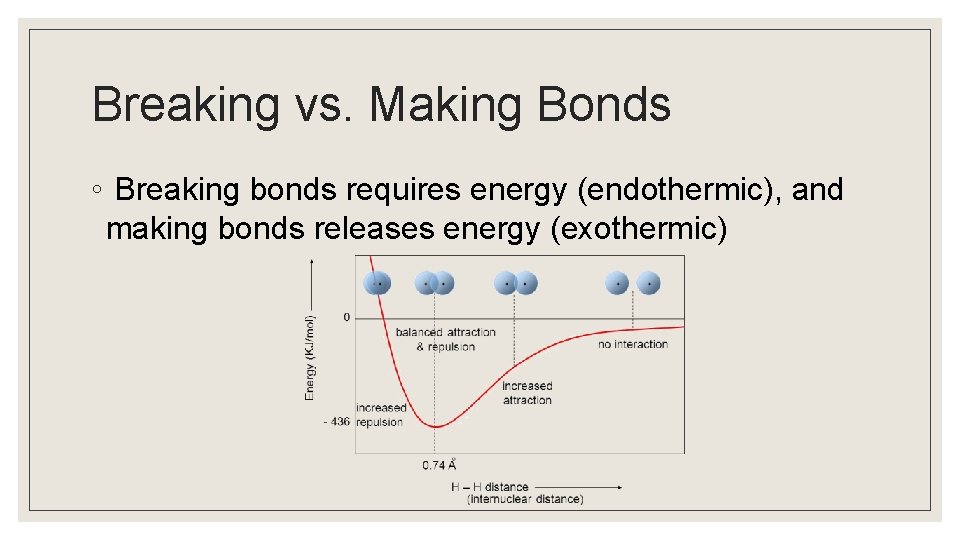
Breaking vs. Making Bonds ◦ Breaking bonds requires energy (endothermic), and making bonds releases energy (exothermic)
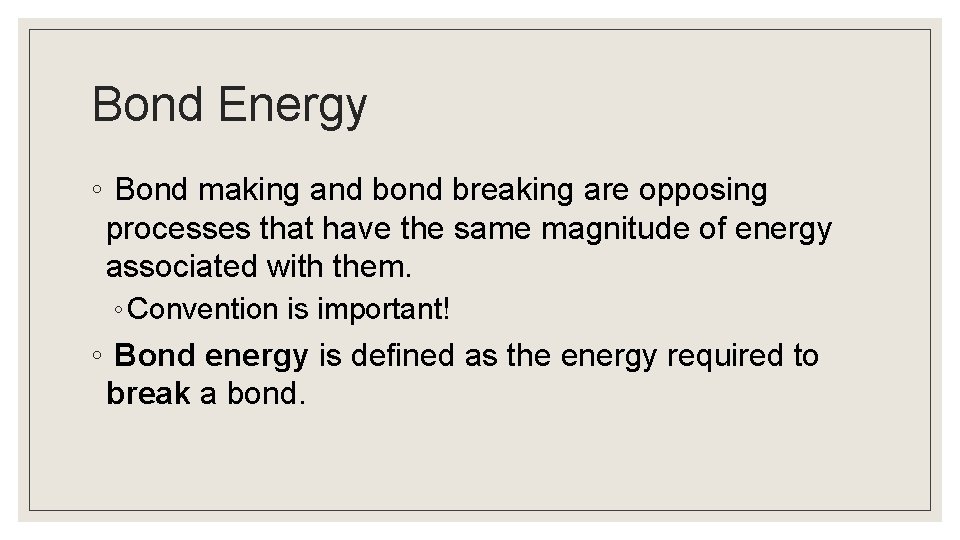
Bond Energy ◦ Bond making and bond breaking are opposing processes that have the same magnitude of energy associated with them. ◦ Convention is important! ◦ Bond energy is defined as the energy required to break a bond.
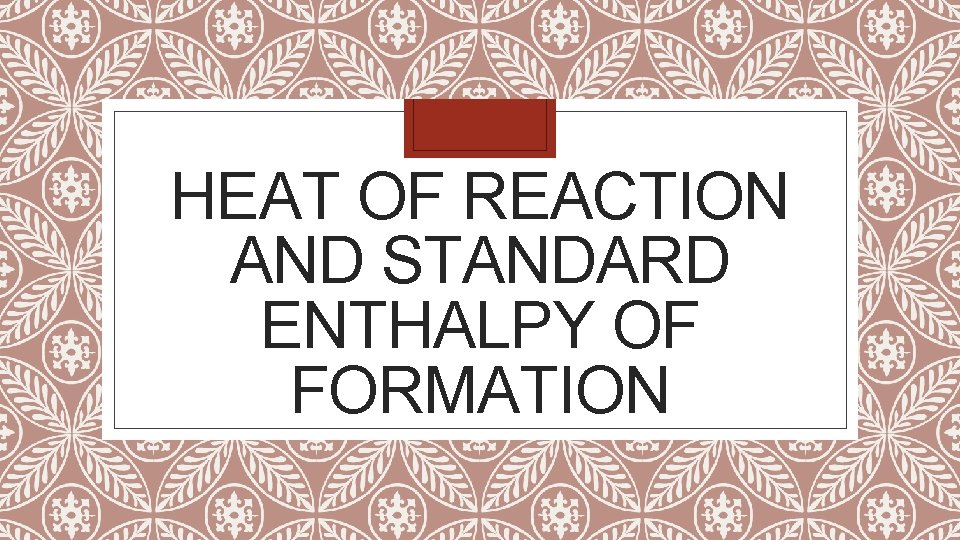
HEAT OF REACTION AND STANDARD ENTHALPY OF FORMATION
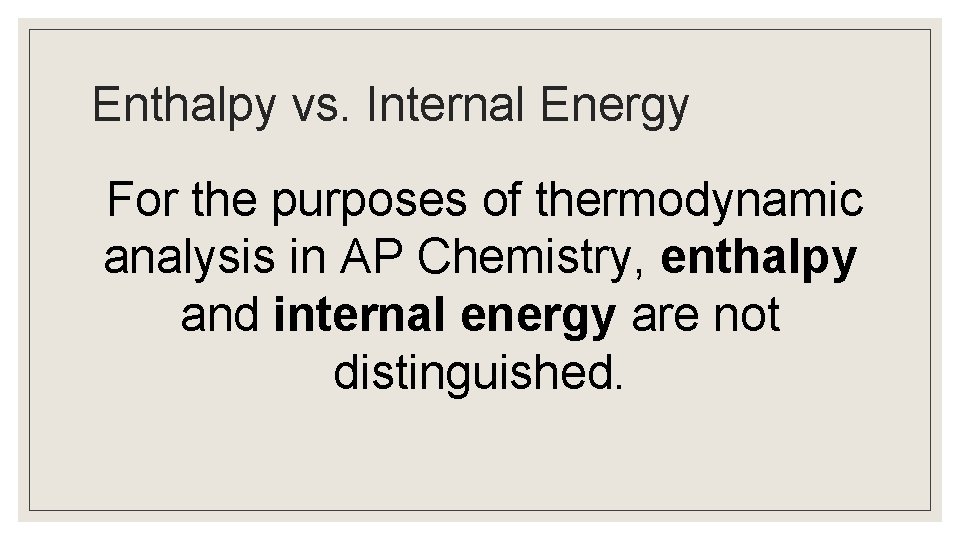
Enthalpy vs. Internal Energy For the purposes of thermodynamic analysis in AP Chemistry, enthalpy and internal energy are not distinguished.
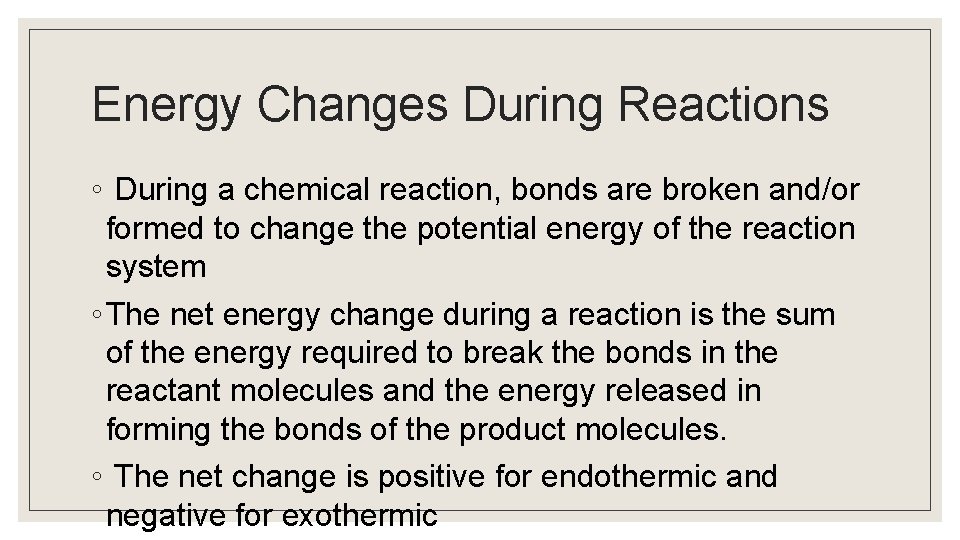
Energy Changes During Reactions ◦ During a chemical reaction, bonds are broken and/or formed to change the potential energy of the reaction system ◦ The net energy change during a reaction is the sum of the energy required to break the bonds in the reactant molecules and the energy released in forming the bonds of the product molecules. ◦ The net change is positive for endothermic and negative for exothermic
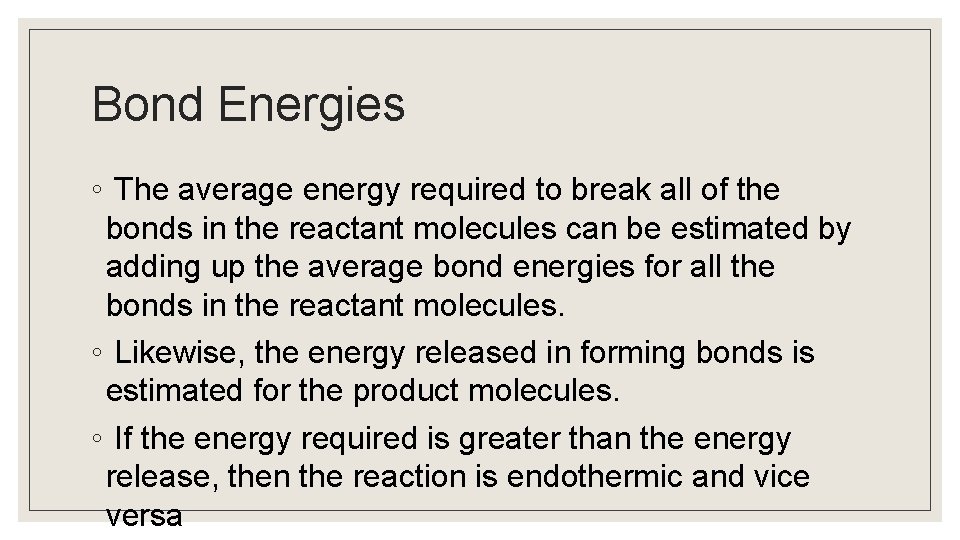
Bond Energies ◦ The average energy required to break all of the bonds in the reactant molecules can be estimated by adding up the average bond energies for all the bonds in the reactant molecules. ◦ Likewise, the energy released in forming bonds is estimated for the product molecules. ◦ If the energy required is greater than the energy release, then the reaction is endothermic and vice versa
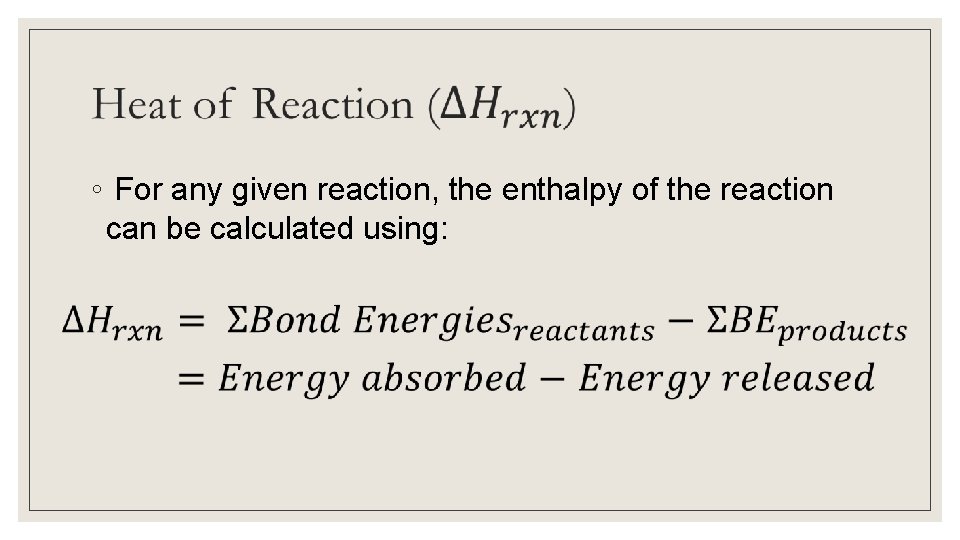
◦ For any given reaction, the enthalpy of the reaction can be calculated using:
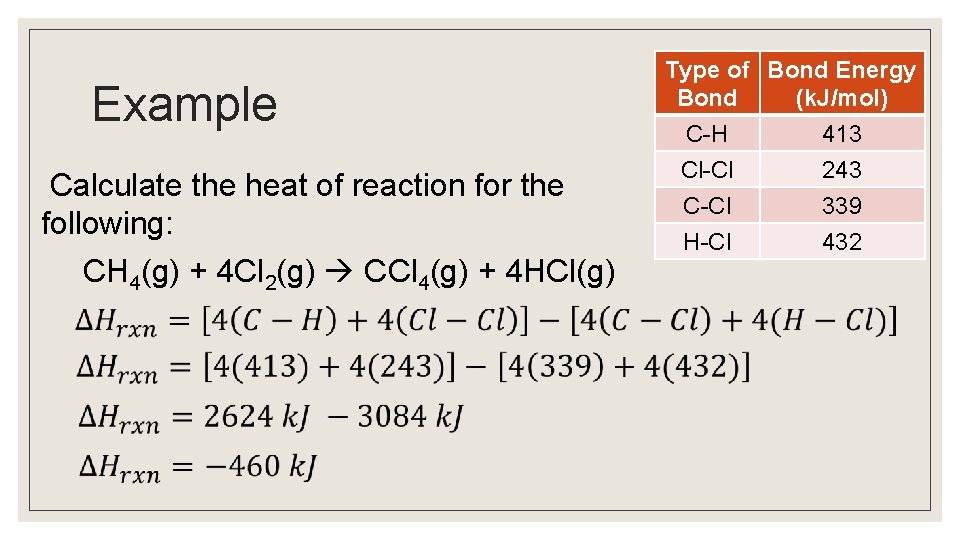
Example Calculate the heat of reaction for the following: CH 4(g) + 4 Cl 2(g) CCl 4(g) + 4 HCl(g) Type of Bond Energy Bond (k. J/mol) C-H 413 Cl-Cl 243 C-Cl H-Cl 339 432
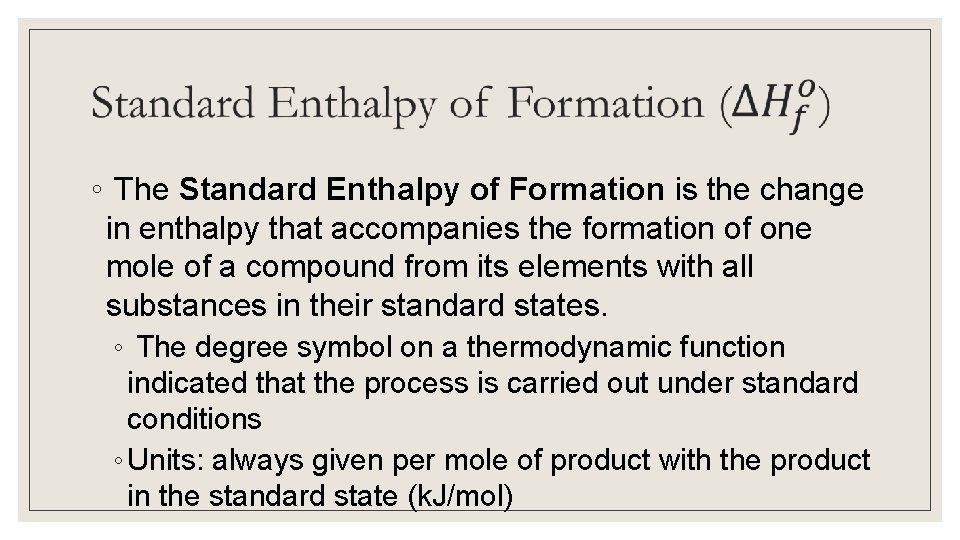
◦ The Standard Enthalpy of Formation is the change in enthalpy that accompanies the formation of one mole of a compound from its elements with all substances in their standard states. ◦ The degree symbol on a thermodynamic function indicated that the process is carried out under standard conditions ◦ Units: always given per mole of product with the product in the standard state (k. J/mol)
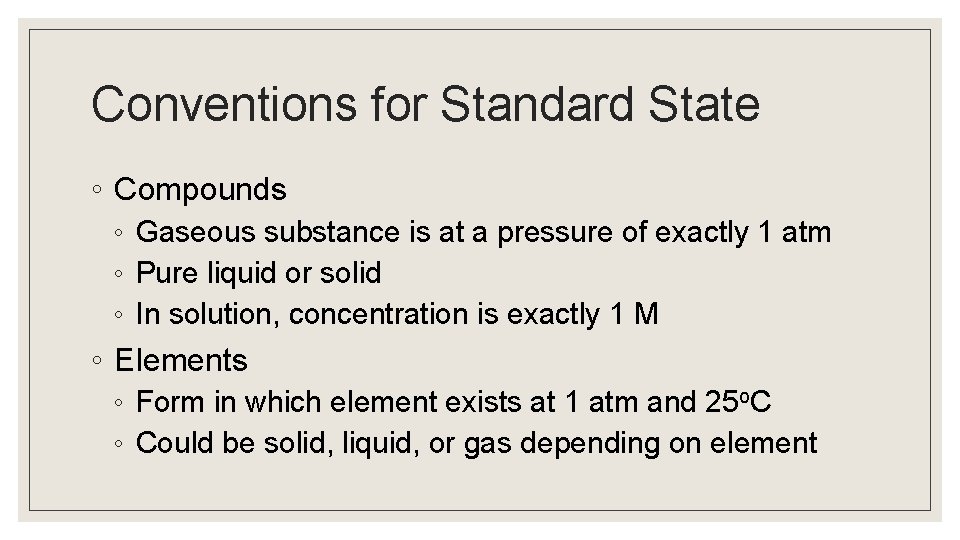
Conventions for Standard State ◦ Compounds ◦ Gaseous substance is at a pressure of exactly 1 atm ◦ Pure liquid or solid ◦ In solution, concentration is exactly 1 M ◦ Elements ◦ Form in which element exists at 1 atm and 25 o. C ◦ Could be solid, liquid, or gas depending on element
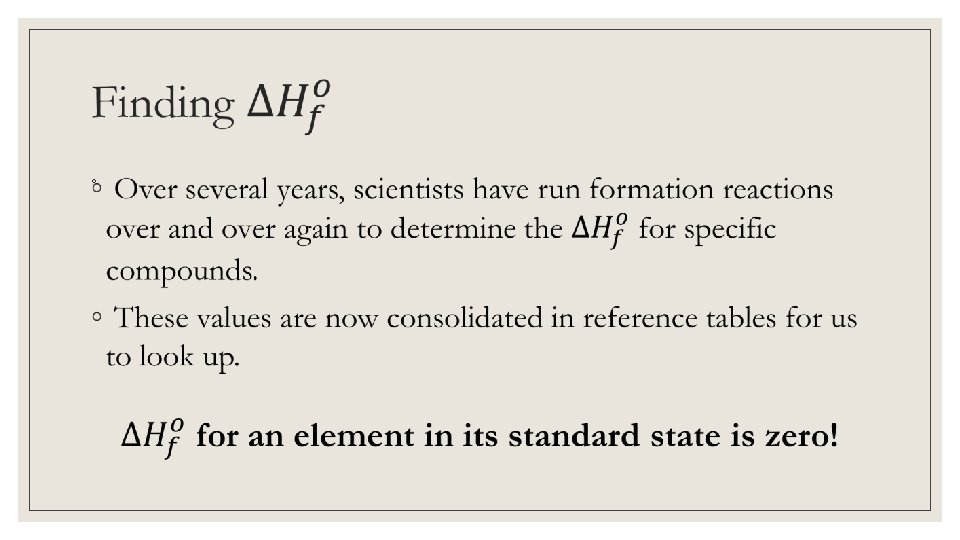
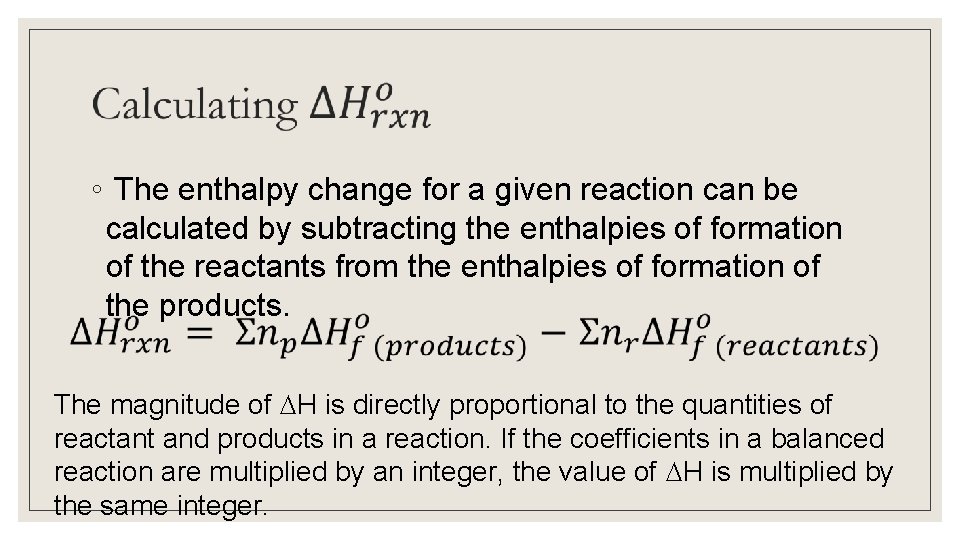
◦ The enthalpy change for a given reaction can be calculated by subtracting the enthalpies of formation of the reactants from the enthalpies of formation of the products. The magnitude of H is directly proportional to the quantities of reactant and products in a reaction. If the coefficients in a balanced reaction are multiplied by an integer, the value of H is multiplied by the same integer.
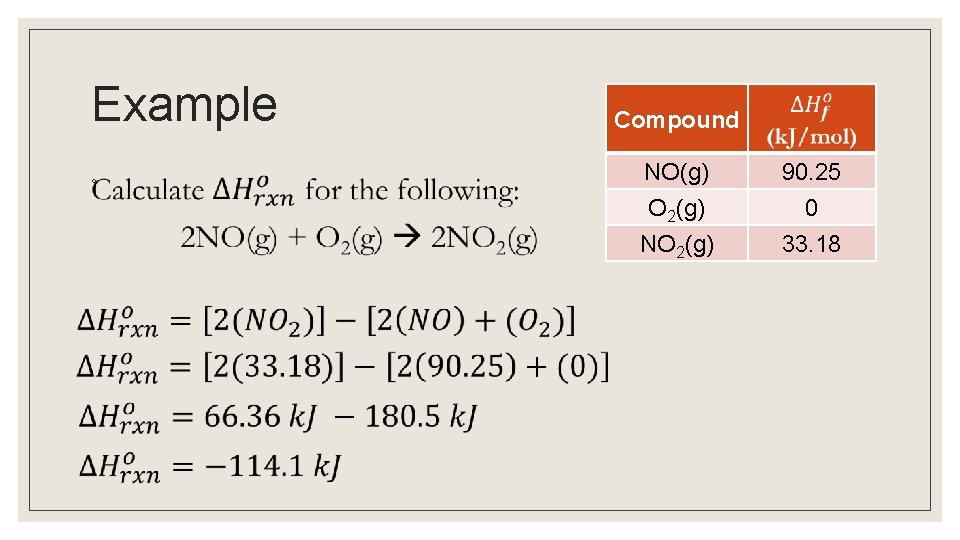
Example ◦ Compound NO(g) O 2(g) 90. 25 0 NO 2(g) 33. 18
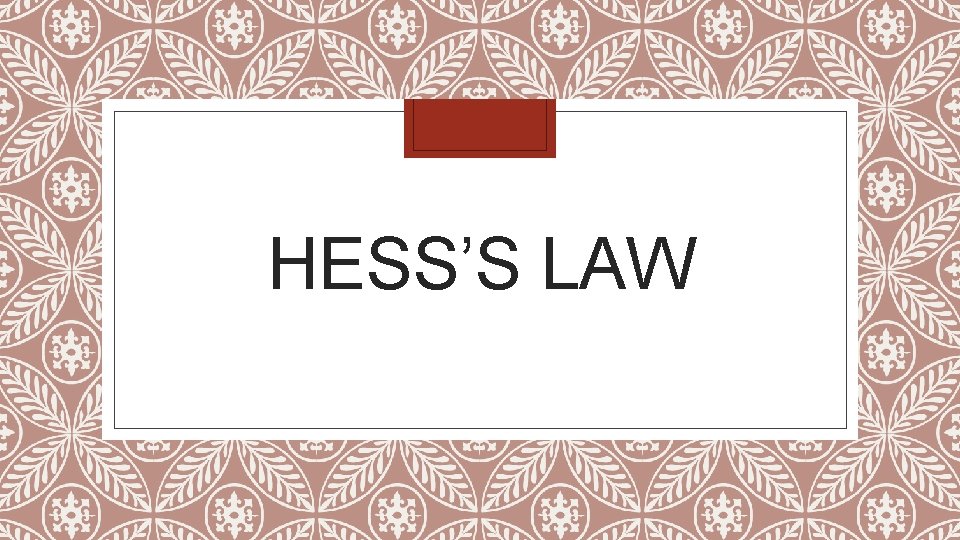
HESS’S LAW
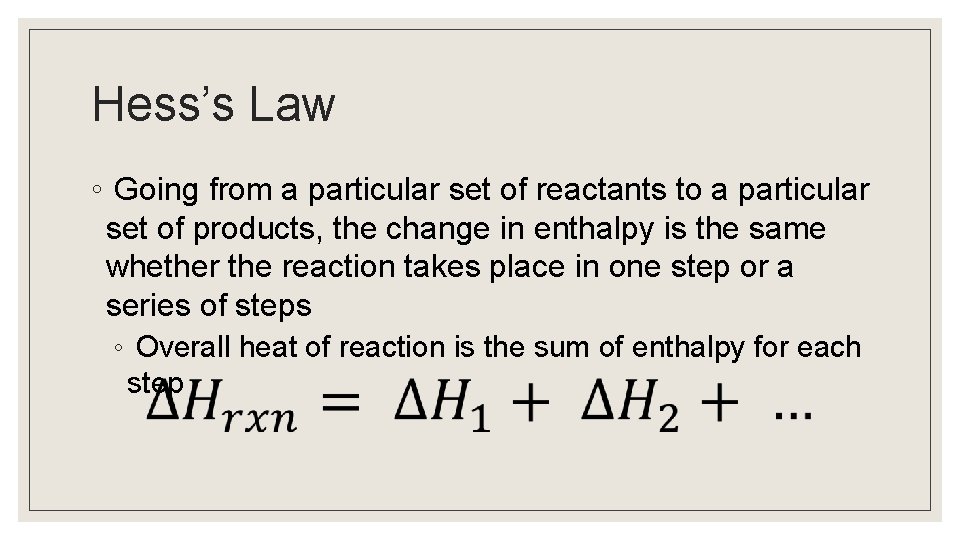
Hess’s Law ◦ Going from a particular set of reactants to a particular set of products, the change in enthalpy is the same whether the reaction takes place in one step or a series of steps ◦ Overall heat of reaction is the sum of enthalpy for each step
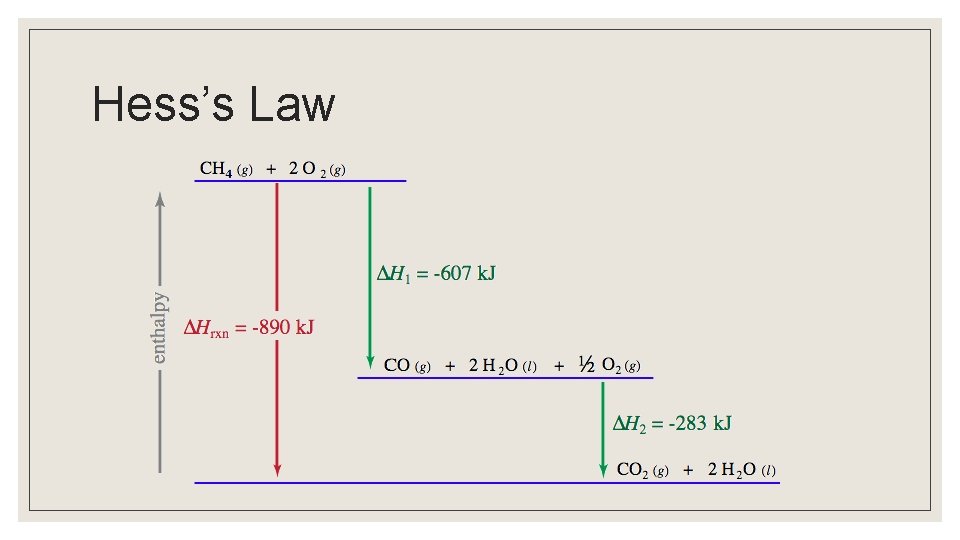
Hess’s Law
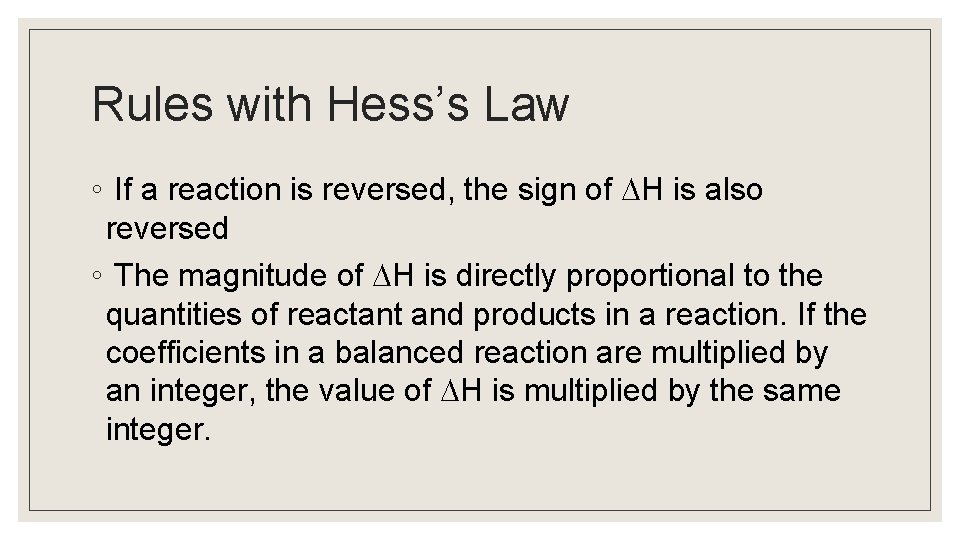
Rules with Hess’s Law ◦ If a reaction is reversed, the sign of H is also reversed ◦ The magnitude of H is directly proportional to the quantities of reactant and products in a reaction. If the coefficients in a balanced reaction are multiplied by an integer, the value of H is multiplied by the same integer.
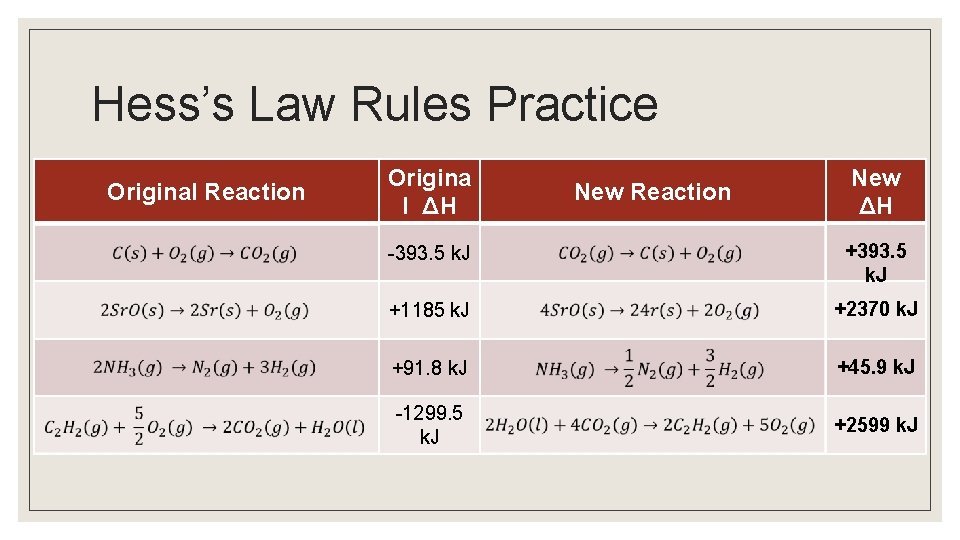
Hess’s Law Rules Practice Original Reaction Origina l ΔH New Reaction New ΔH -393. 5 k. J +1185 k. J +2370 k. J +91. 8 k. J +45. 9 k. J -1299. 5 k. J +2599 k. J
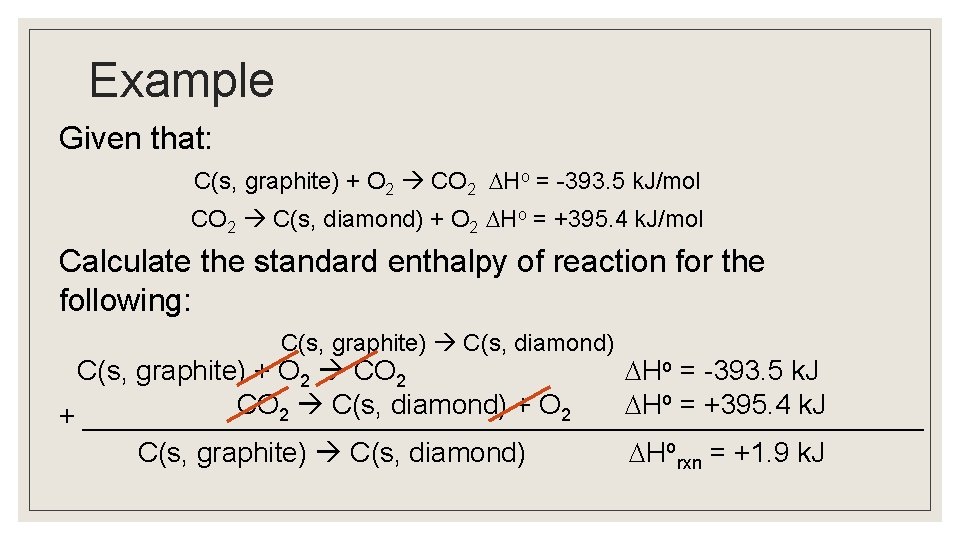
Example Given that: C(s, graphite) + O 2 CO 2 Ho = -393. 5 k. J/mol CO 2 C(s, diamond) + O 2 Ho = +395. 4 k. J/mol Calculate the standard enthalpy of reaction for the following: C(s, graphite) C(s, diamond) C(s, graphite) + O 2 CO 2 Ho = -393. 5 k. J o = +395. 4 k. J CO C(s, diamond) + O H 2 2 + ___________________________ C(s, graphite) C(s, diamond) Horxn = +1. 9 k. J
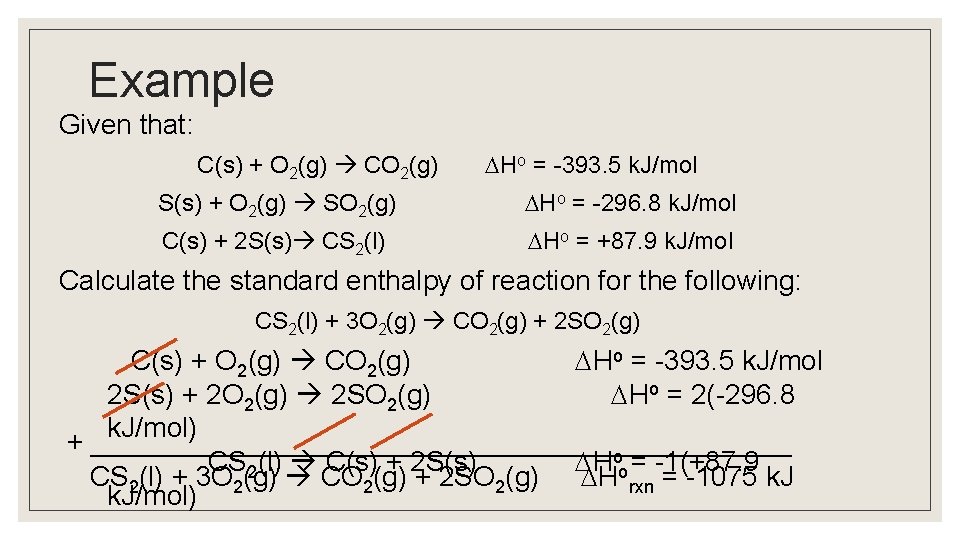
Example Given that: Ho = -393. 5 k. J/mol C(s) + O 2(g) CO 2(g) S(s) + O 2(g) SO 2(g) C(s) + 2 S(s) CS 2(l) Ho = -296. 8 k. J/mol Ho = +87. 9 k. J/mol Calculate the standard enthalpy of reaction for the following: CS 2(l) + 3 O 2(g) CO 2(g) + 2 SO 2(g) C(s) + O 2(g) CO 2(g) Ho = -393. 5 k. J/mol 2 S(s) + 2 O 2(g) 2 SO 2(g) Ho = 2(-296. 8 k. J/mol) + _______________________ CS 2(l) C(s) + 2 S(s) Hoo = -1(+87. 9 CS 2(l) + 3 O 2(g) CO 2(g) + 2 SO 2(g) H rxn = -1075 k. J/mol)
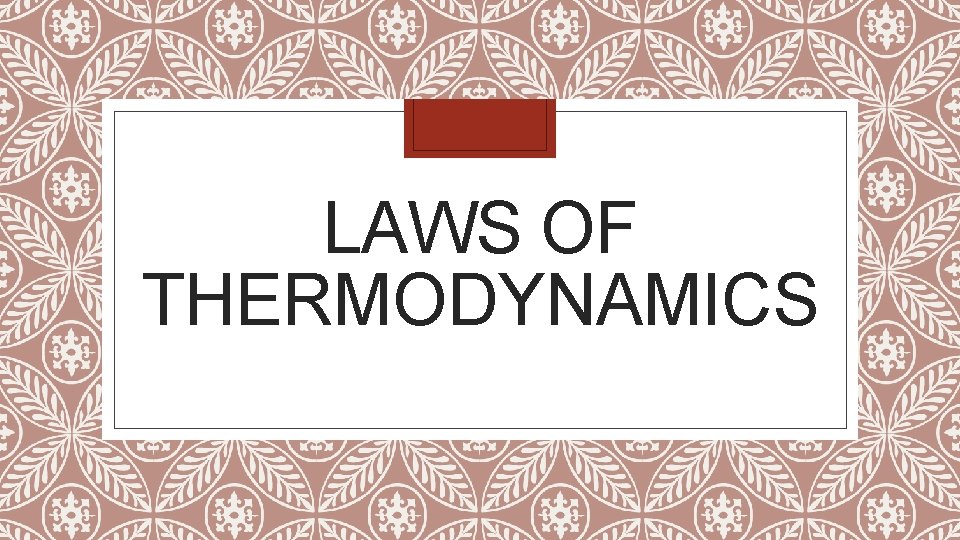
LAWS OF THERMODYNAMICS
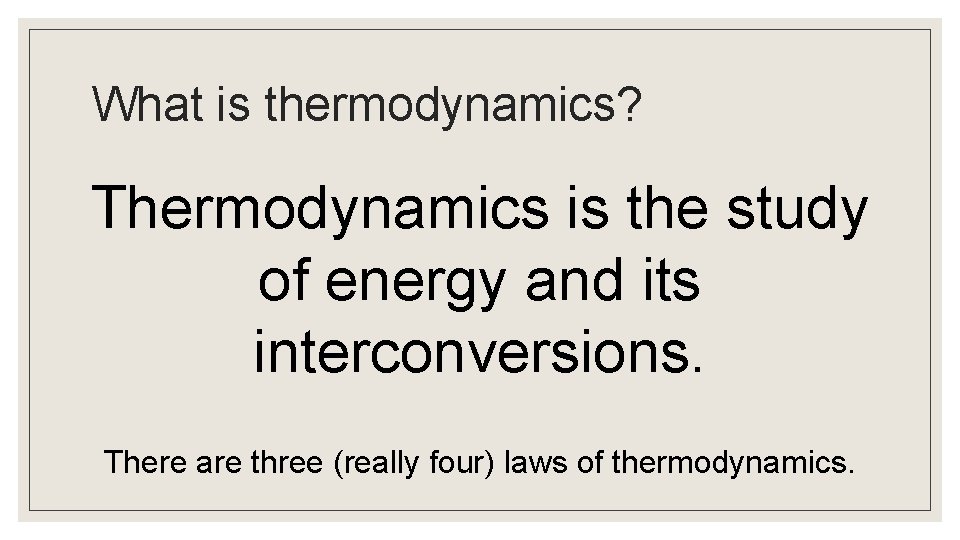
What is thermodynamics? Thermodynamics is the study of energy and its interconversions. There are three (really four) laws of thermodynamics.
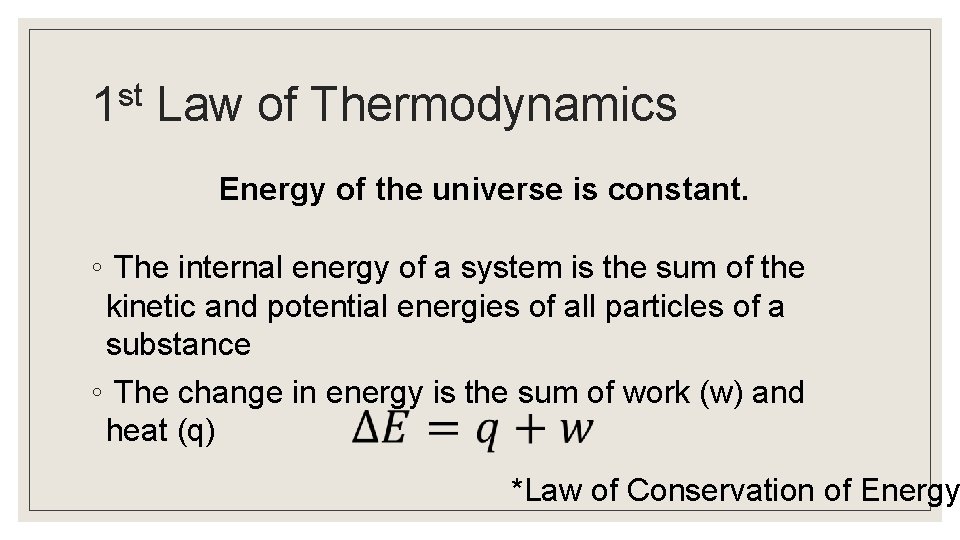
st 1 Law of Thermodynamics Energy of the universe is constant. ◦ The internal energy of a system is the sum of the kinetic and potential energies of all particles of a substance ◦ The change in energy is the sum of work (w) and heat (q) *Law of Conservation of Energy
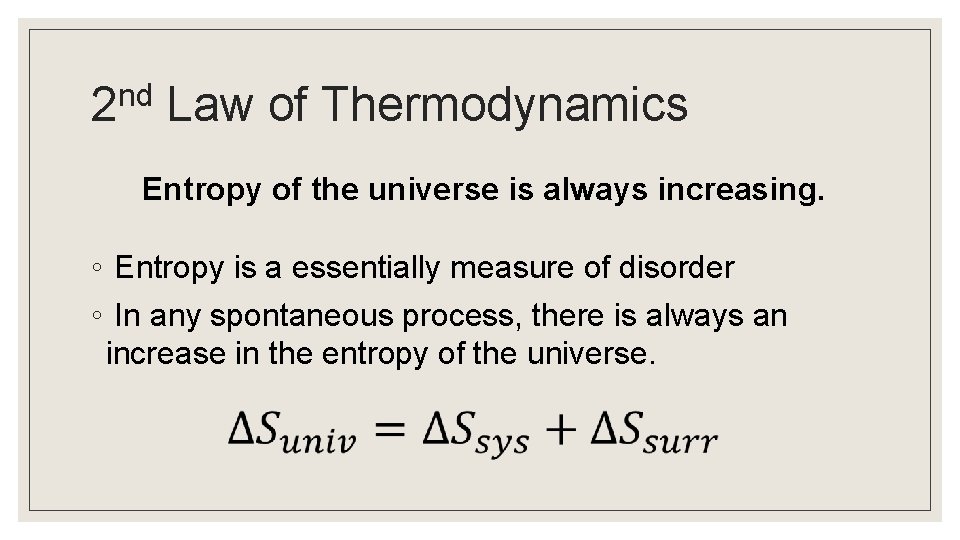
nd 2 Law of Thermodynamics Entropy of the universe is always increasing. ◦ Entropy is a essentially measure of disorder ◦ In any spontaneous process, there is always an increase in the entropy of the universe.
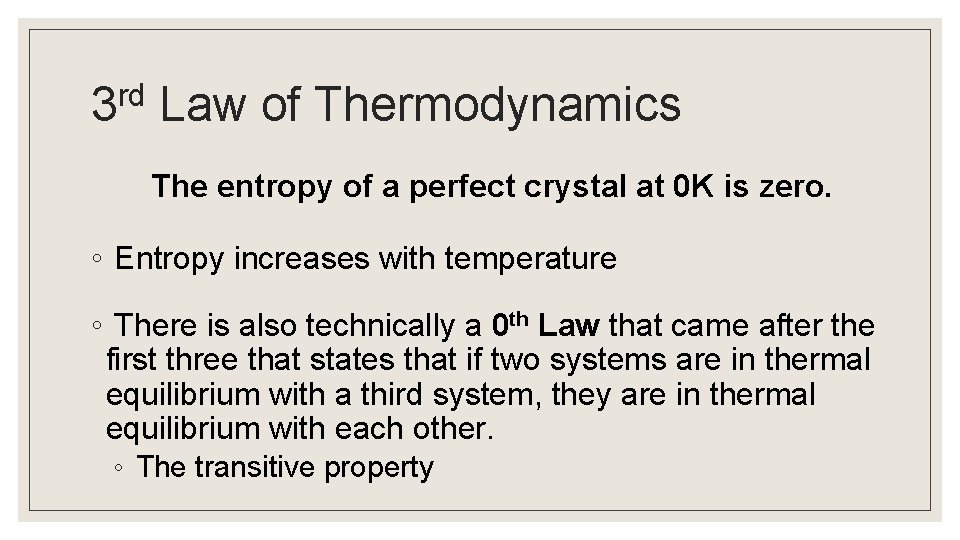
rd 3 Law of Thermodynamics The entropy of a perfect crystal at 0 K is zero. ◦ Entropy increases with temperature ◦ There is also technically a 0 th Law that came after the first three that states that if two systems are in thermal equilibrium with a third system, they are in thermal equilibrium with each other. ◦ The transitive property
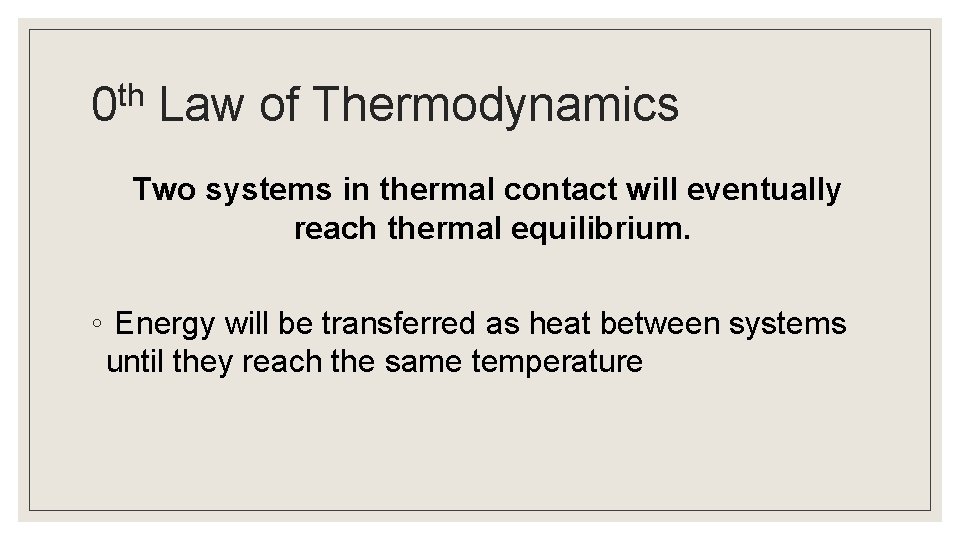
th 0 Law of Thermodynamics Two systems in thermal contact will eventually reach thermal equilibrium. ◦ Energy will be transferred as heat between systems until they reach the same temperature
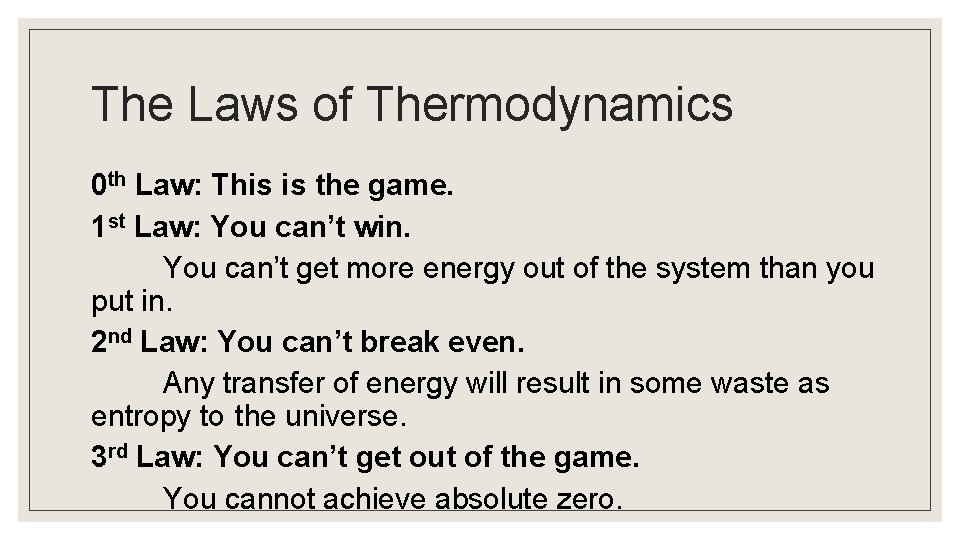
The Laws of Thermodynamics 0 th Law: This is the game. 1 st Law: You can’t win. You can’t get more energy out of the system than you put in. 2 nd Law: You can’t break even. Any transfer of energy will result in some waste as entropy to the universe. 3 rd Law: You can’t get out of the game. You cannot achieve absolute zero.
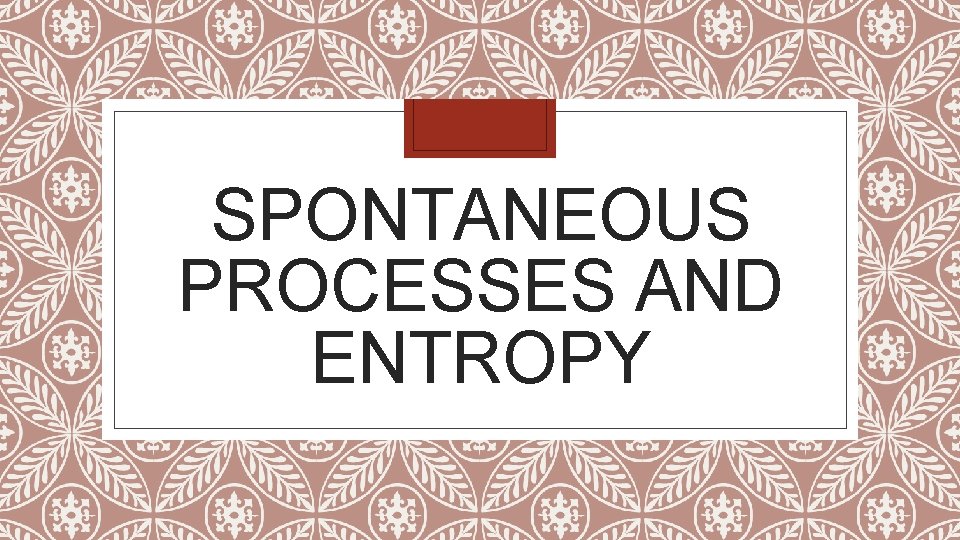
SPONTANEOUS PROCESSES AND ENTROPY
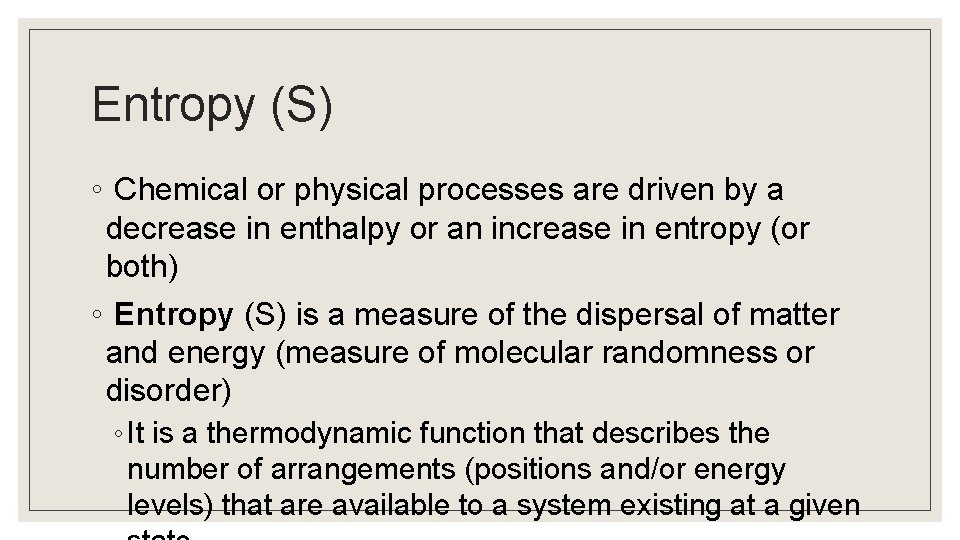
Entropy (S) ◦ Chemical or physical processes are driven by a decrease in enthalpy or an increase in entropy (or both) ◦ Entropy (S) is a measure of the dispersal of matter and energy (measure of molecular randomness or disorder) ◦ It is a thermodynamic function that describes the number of arrangements (positions and/or energy levels) that are available to a system existing at a given
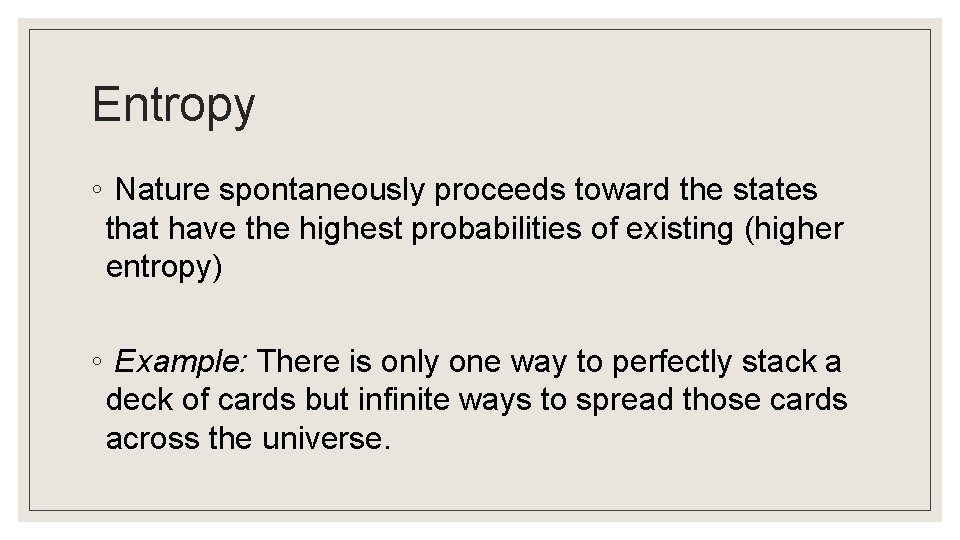
Entropy ◦ Nature spontaneously proceeds toward the states that have the highest probabilities of existing (higher entropy) ◦ Example: There is only one way to perfectly stack a deck of cards but infinite ways to spread those cards across the universe.
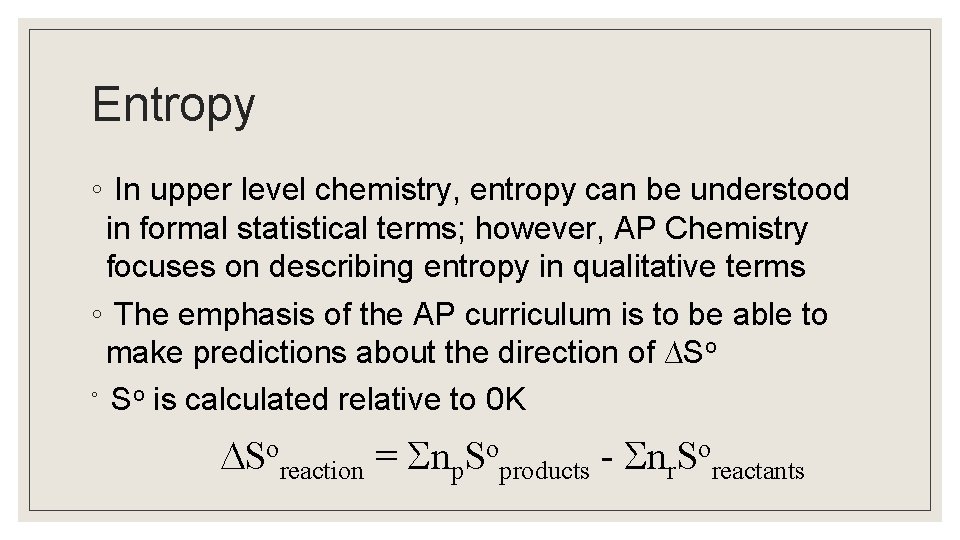
Entropy ◦ In upper level chemistry, entropy can be understood in formal statistical terms; however, AP Chemistry focuses on describing entropy in qualitative terms ◦ The emphasis of the AP curriculum is to be able to make predictions about the direction of ∆So ◦ So is calculated relative to 0 K Soreaction = np. Soproducts - nr. Soreactants
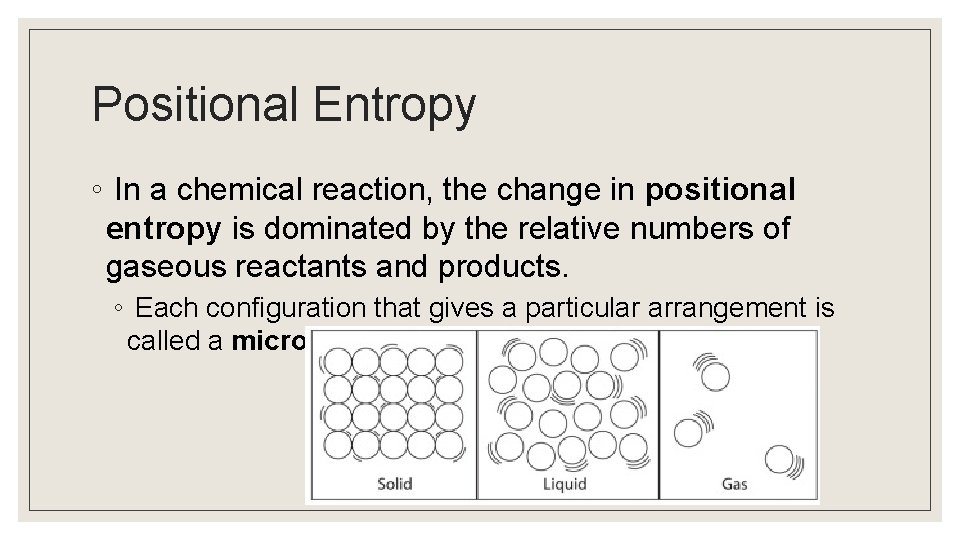
Positional Entropy ◦ In a chemical reaction, the change in positional entropy is dominated by the relative numbers of gaseous reactants and products. ◦ Each configuration that gives a particular arrangement is called a microstate
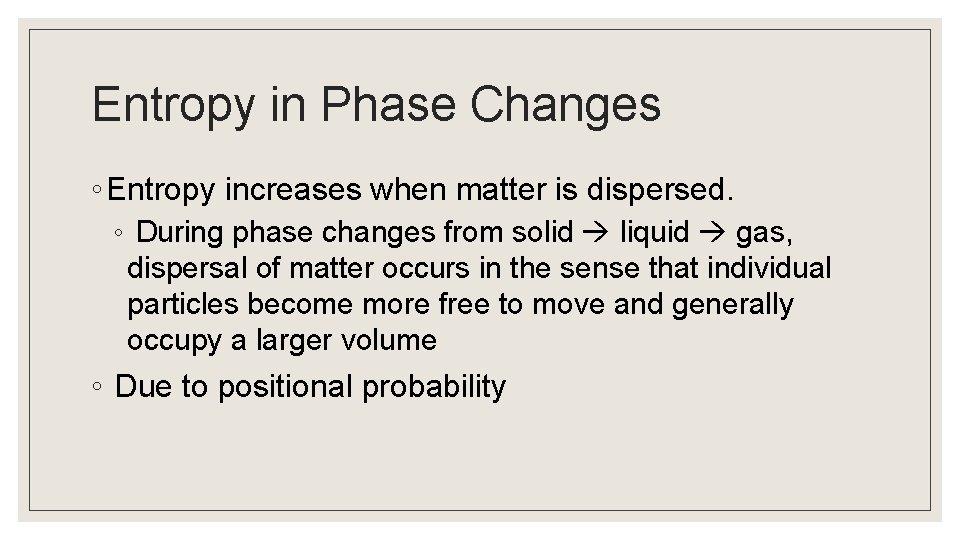
Entropy in Phase Changes ◦ Entropy increases when matter is dispersed. ◦ During phase changes from solid liquid gas, dispersal of matter occurs in the sense that individual particles become more free to move and generally occupy a larger volume ◦ Due to positional probability
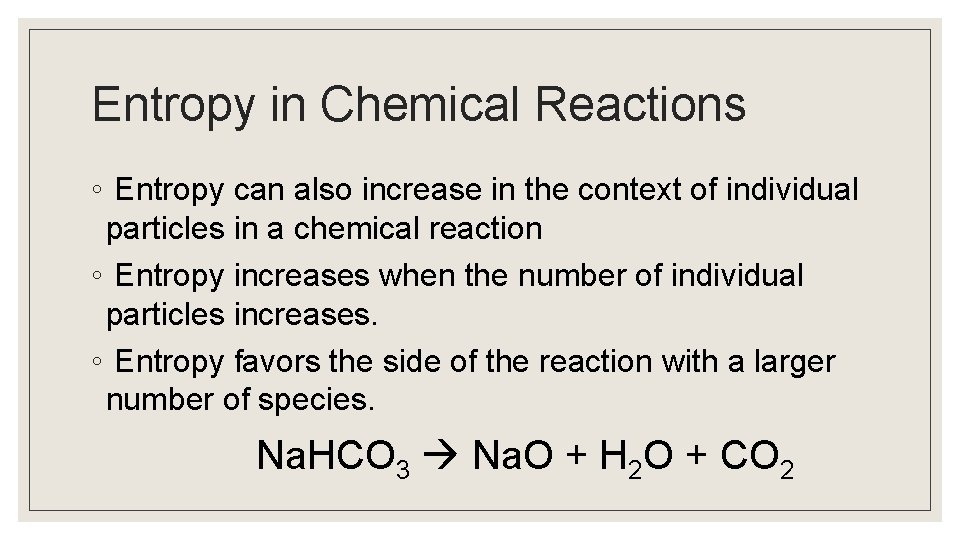
Entropy in Chemical Reactions ◦ Entropy can also increase in the context of individual particles in a chemical reaction ◦ Entropy increases when the number of individual particles increases. ◦ Entropy favors the side of the reaction with a larger number of species. Na. HCO 3 Na. O + H 2 O + CO 2
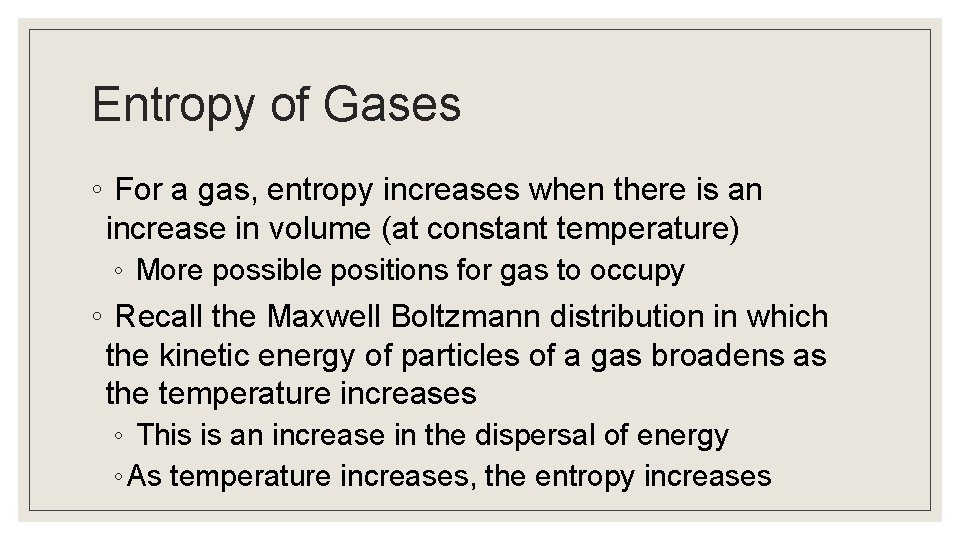
Entropy of Gases ◦ For a gas, entropy increases when there is an increase in volume (at constant temperature) ◦ More possible positions for gas to occupy ◦ Recall the Maxwell Boltzmann distribution in which the kinetic energy of particles of a gas broadens as the temperature increases ◦ This is an increase in the dispersal of energy ◦ As temperature increases, the entropy increases
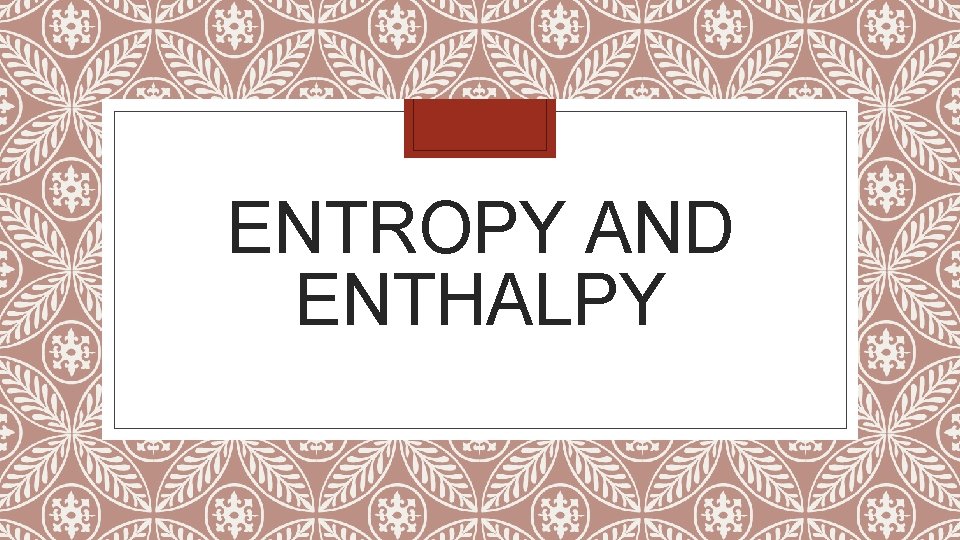
ENTROPY AND ENTHALPY
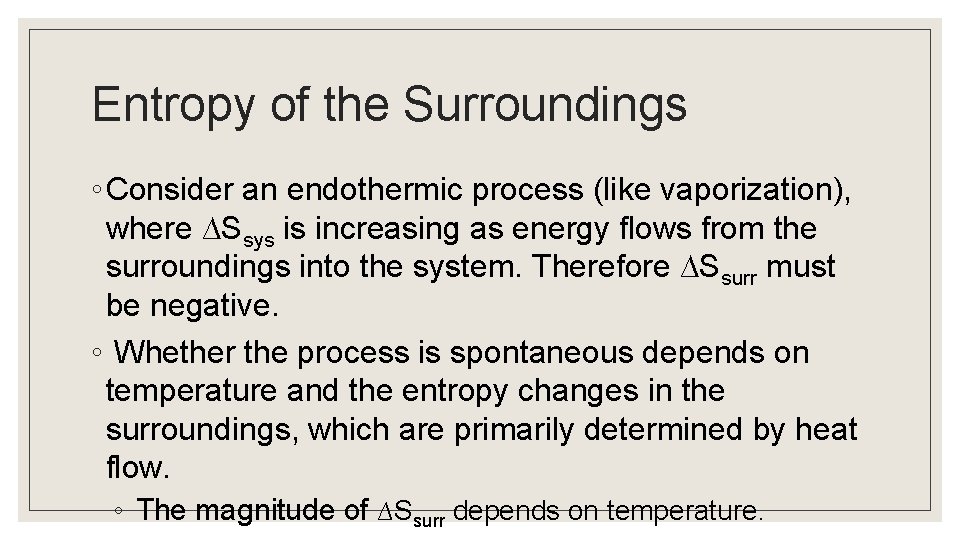
Entropy of the Surroundings ◦ Consider an endothermic process (like vaporization), where ∆Ssys is increasing as energy flows from the surroundings into the system. Therefore ∆Ssurr must be negative. ◦ Whether the process is spontaneous depends on temperature and the entropy changes in the surroundings, which are primarily determined by heat flow. ◦ The magnitude of ∆Ssurr depends on temperature.
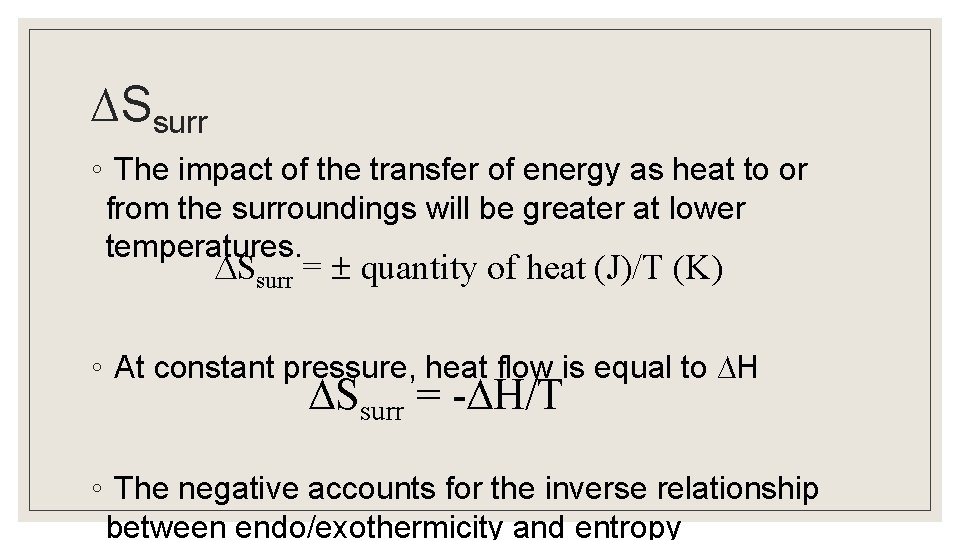
∆Ssurr ◦ The impact of the transfer of energy as heat to or from the surroundings will be greater at lower temperatures. Ssurr = quantity of heat (J)/T (K) ◦ At constant pressure, heat flow is equal to ∆H Ssurr = - H/T ◦ The negative accounts for the inverse relationship between endo/exothermicity and entropy
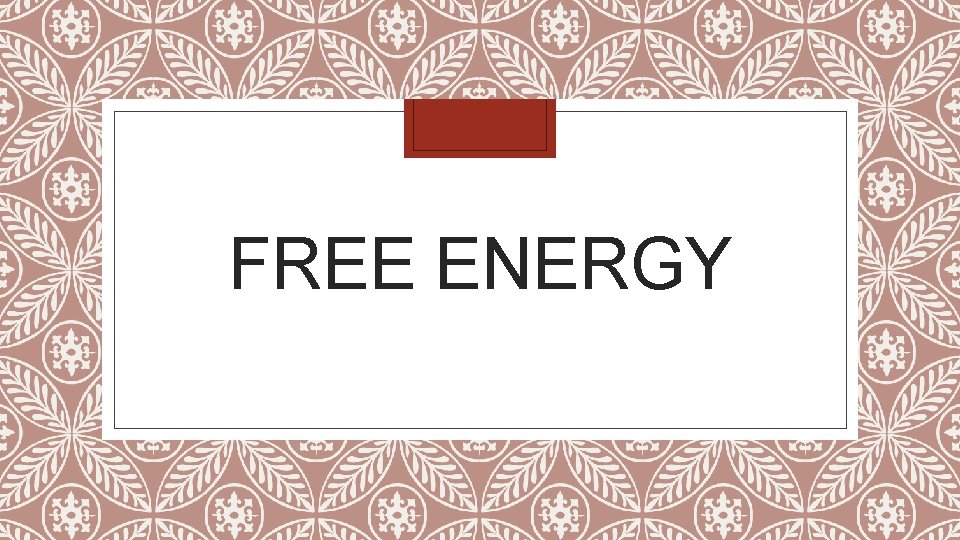
FREE ENERGY
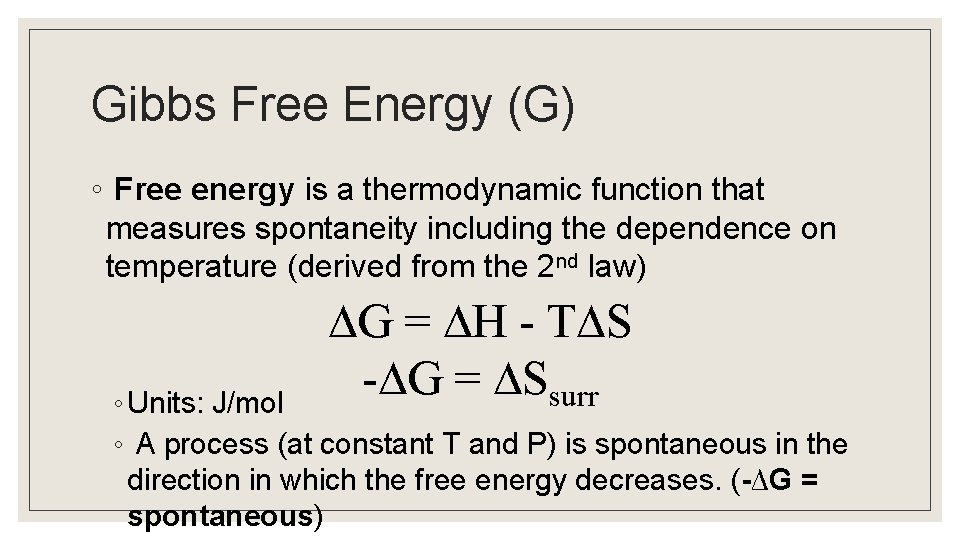
Gibbs Free Energy (G) ◦ Free energy is a thermodynamic function that measures spontaneity including the dependence on temperature (derived from the 2 nd law) G = H - T S - G = Ssurr ◦ Units: J/mol ◦ A process (at constant T and P) is spontaneous in the direction in which the free energy decreases. (-∆G = spontaneous)
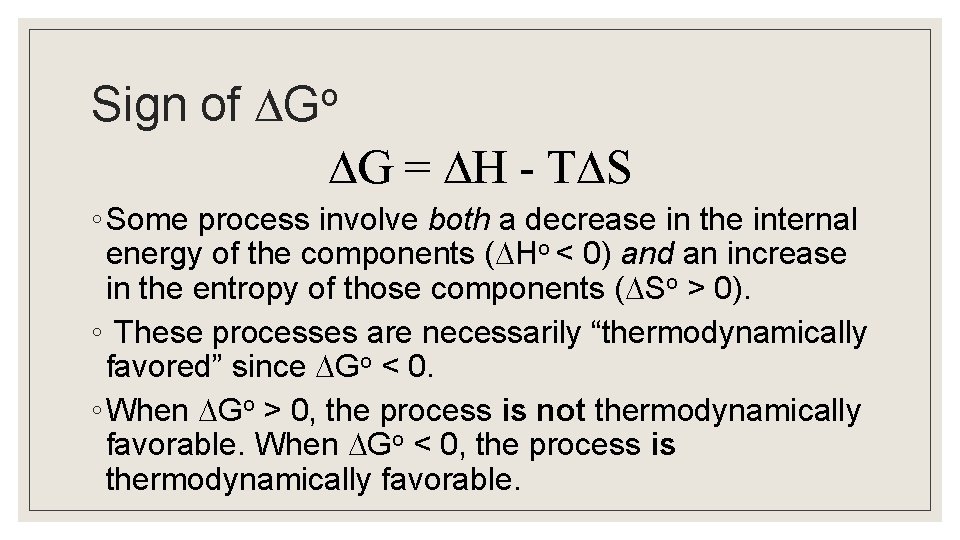
o Sign of G G = H - T S ◦ Some process involve both a decrease in the internal energy of the components ( Ho < 0) and an increase in the entropy of those components ( So > 0). ◦ These processes are necessarily “thermodynamically favored” since Go < 0. ◦ When Go > 0, the process is not thermodynamically favorable. When Go < 0, the process is thermodynamically favorable.
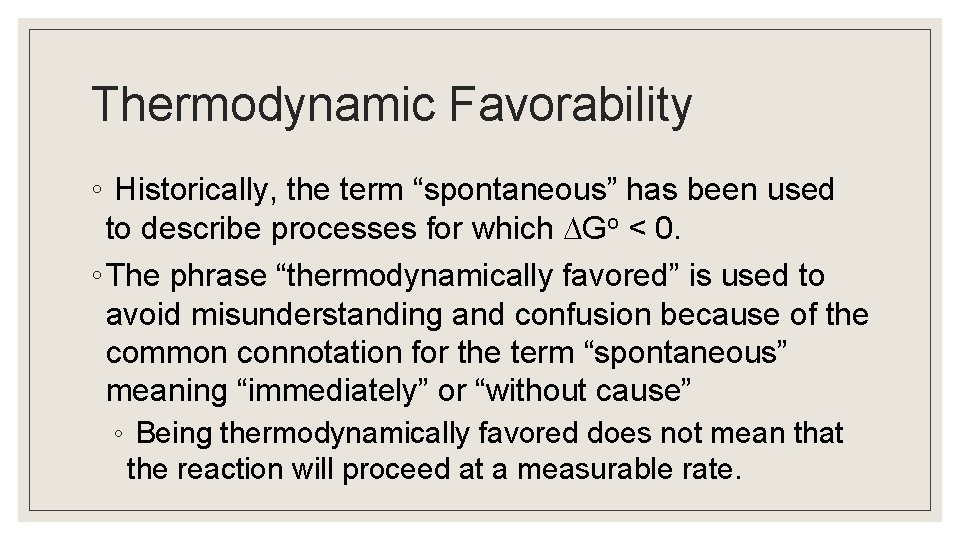
Thermodynamic Favorability ◦ Historically, the term “spontaneous” has been used to describe processes for which Go < 0. ◦ The phrase “thermodynamically favored” is used to avoid misunderstanding and confusion because of the common connotation for the term “spontaneous” meaning “immediately” or “without cause” ◦ Being thermodynamically favored does not mean that the reaction will proceed at a measurable rate.
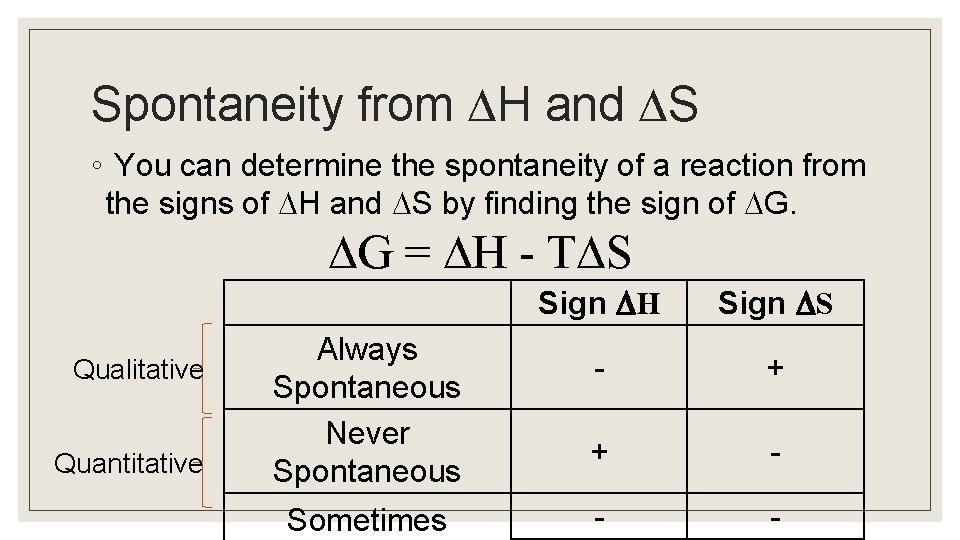
Spontaneity from H and S ◦ You can determine the spontaneity of a reaction from the signs of H and S by finding the sign of G. G = H - T S Qualitative Quantitative Always Spontaneous Never Spontaneous Sometimes Sign H Sign S - + + - - -
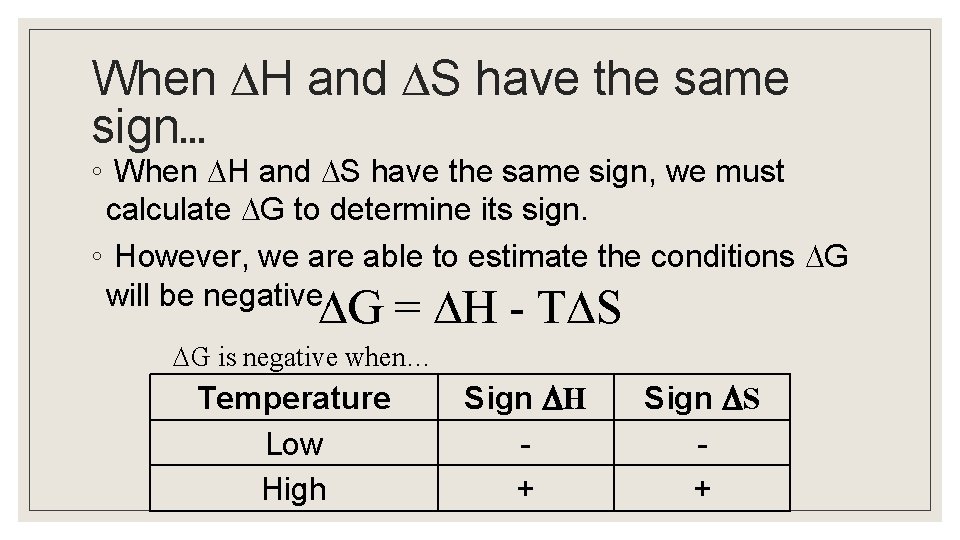
When H and S have the same sign… ◦ When H and S have the same sign, we must calculate G to determine its sign. ◦ However, we are able to estimate the conditions G will be negative. G = H - T S G is negative when… Temperature Low High Sign H + Sign S +
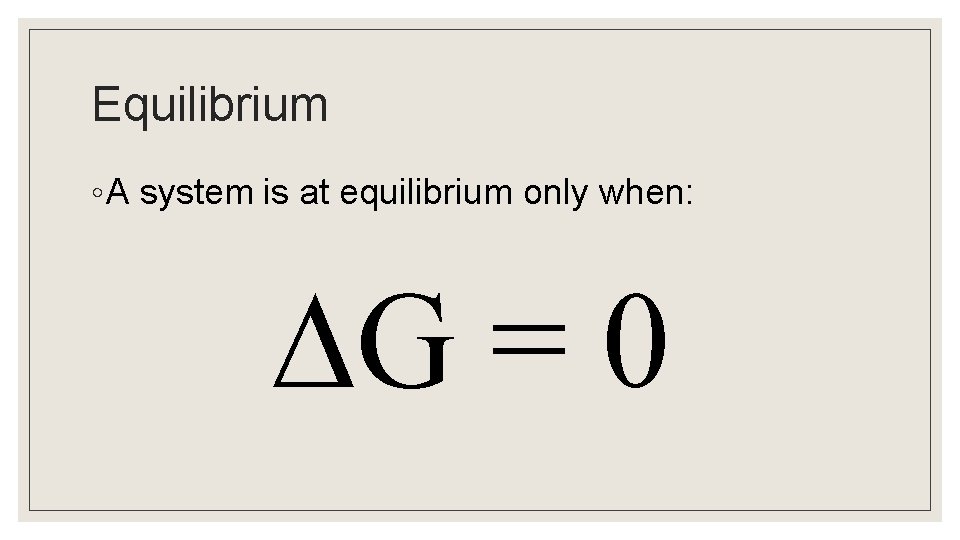
Equilibrium ◦ A system is at equilibrium only when: G = 0
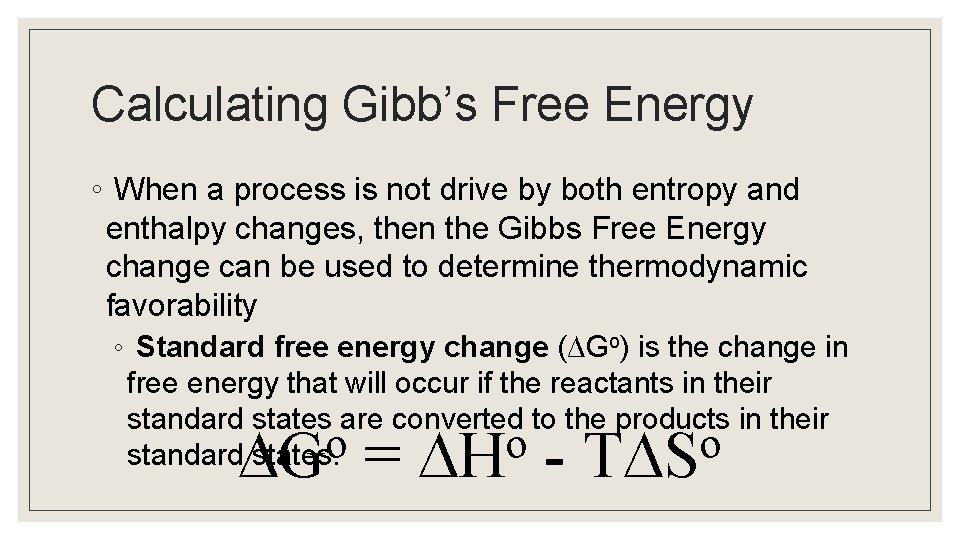
Calculating Gibb’s Free Energy ◦ When a process is not drive by both entropy and enthalpy changes, then the Gibbs Free Energy change can be used to determine thermodynamic favorability ◦ Standard free energy change (∆Go) is the change in free energy that will occur if the reactants in their standard states are converted to the products in their o o standard states. o G = H - T S
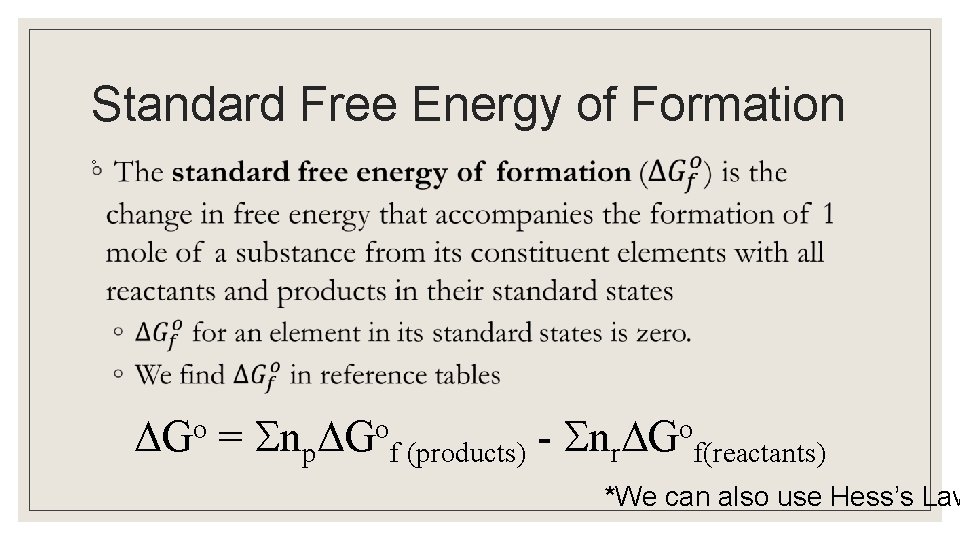
Standard Free Energy of Formation ◦ Go = np Gof (products) - nr Gof(reactants) *We can also use Hess’s Law
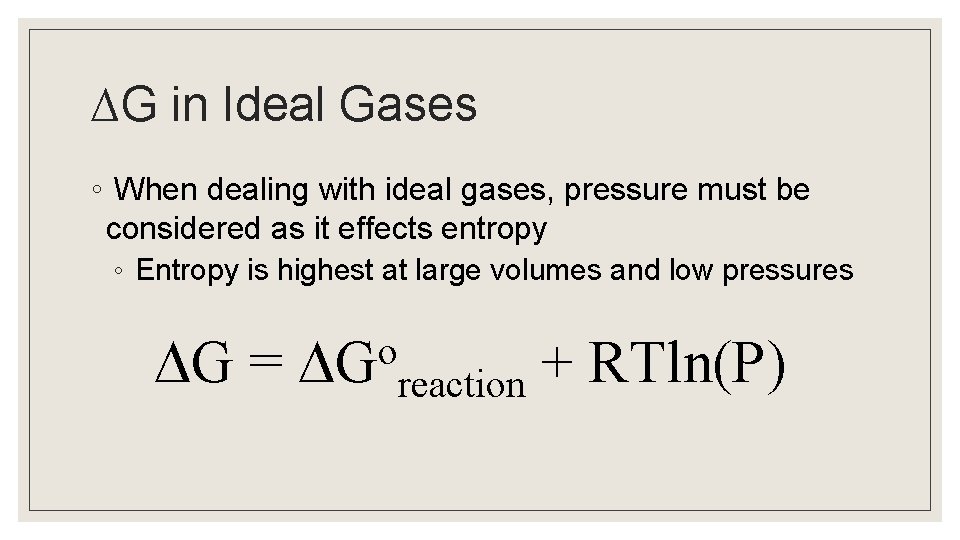
∆G in Ideal Gases ◦ When dealing with ideal gases, pressure must be considered as it effects entropy ◦ Entropy is highest at large volumes and low pressures G = o G + RTln(P) reaction
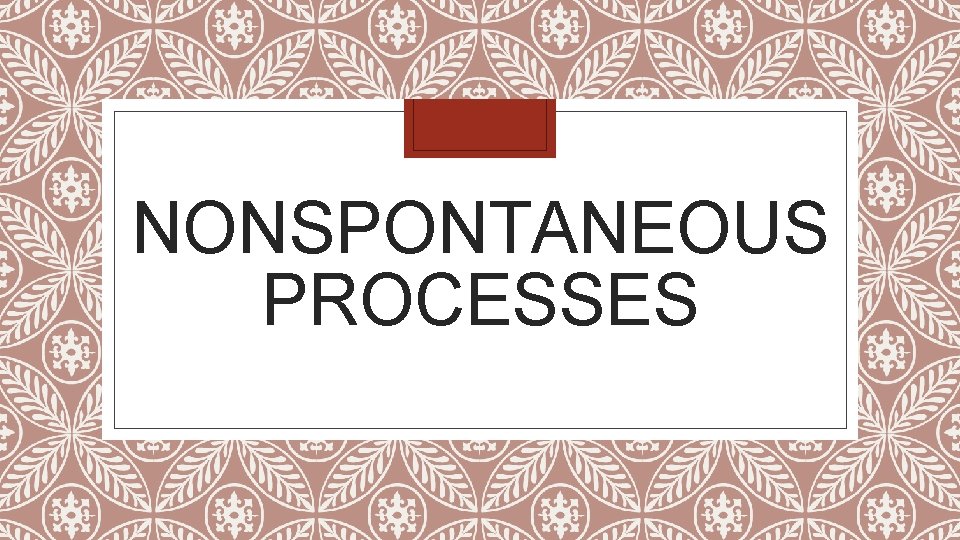
NONSPONTANEOUS PROCESSES
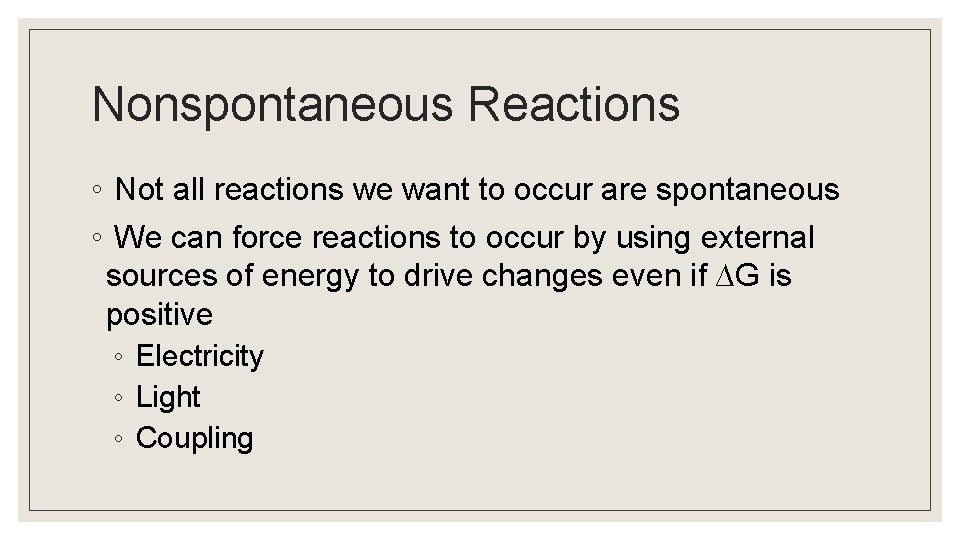
Nonspontaneous Reactions ◦ Not all reactions we want to occur are spontaneous ◦ We can force reactions to occur by using external sources of energy to drive changes even if ∆G is positive ◦ Electricity ◦ Light ◦ Coupling
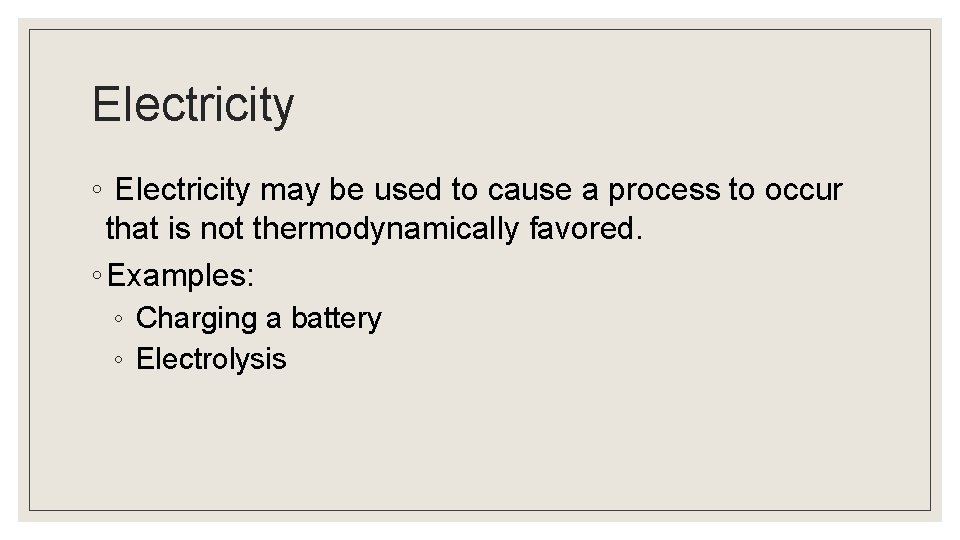
Electricity ◦ Electricity may be used to cause a process to occur that is not thermodynamically favored. ◦ Examples: ◦ Charging a battery ◦ Electrolysis
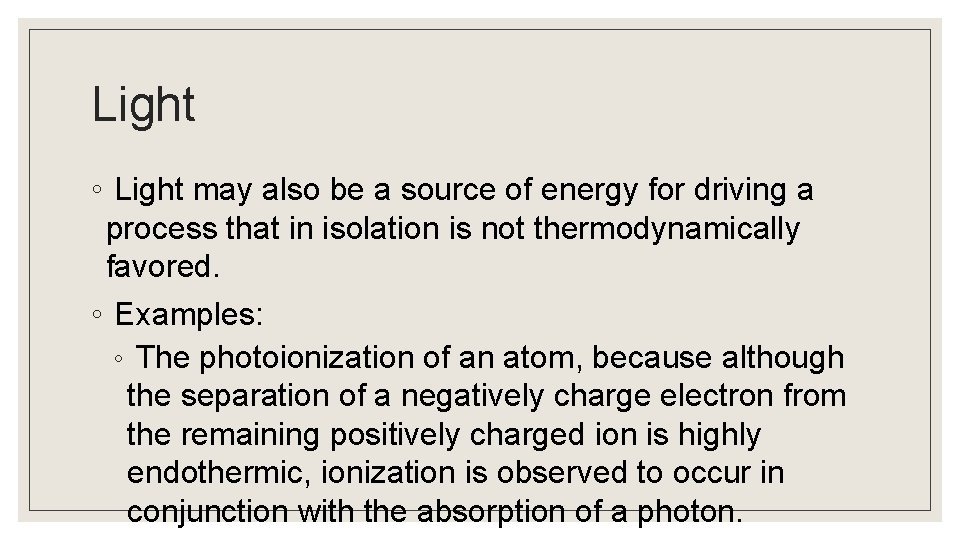
Light ◦ Light may also be a source of energy for driving a process that in isolation is not thermodynamically favored. ◦ Examples: ◦ The photoionization of an atom, because although the separation of a negatively charge electron from the remaining positively charged ion is highly endothermic, ionization is observed to occur in conjunction with the absorption of a photon.
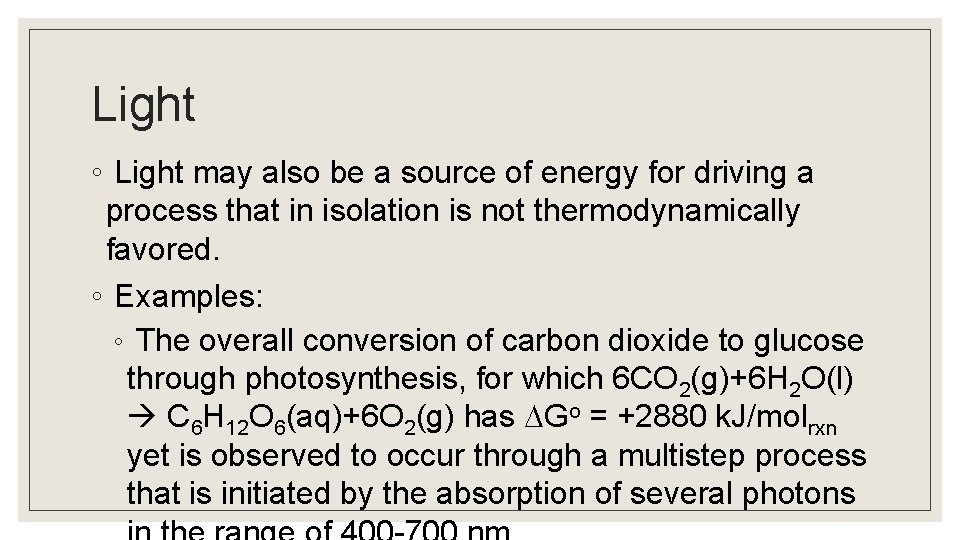
Light ◦ Light may also be a source of energy for driving a process that in isolation is not thermodynamically favored. ◦ Examples: ◦ The overall conversion of carbon dioxide to glucose through photosynthesis, for which 6 CO 2(g)+6 H 2 O(l) C 6 H 12 O 6(aq)+6 O 2(g) has Go = +2880 k. J/molrxn yet is observed to occur through a multistep process that is initiated by the absorption of several photons
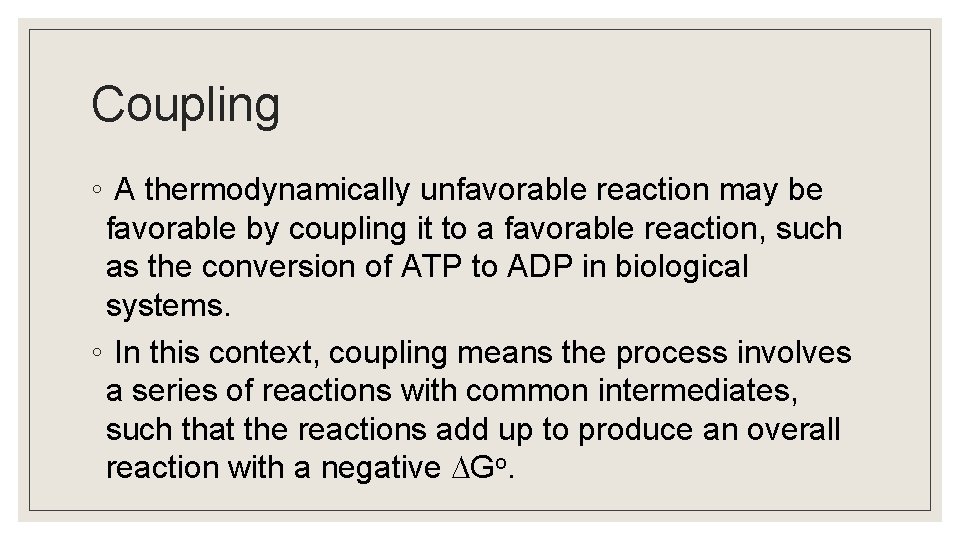
Coupling ◦ A thermodynamically unfavorable reaction may be favorable by coupling it to a favorable reaction, such as the conversion of ATP to ADP in biological systems. ◦ In this context, coupling means the process involves a series of reactions with common intermediates, such that the reactions add up to produce an overall reaction with a negative Go.
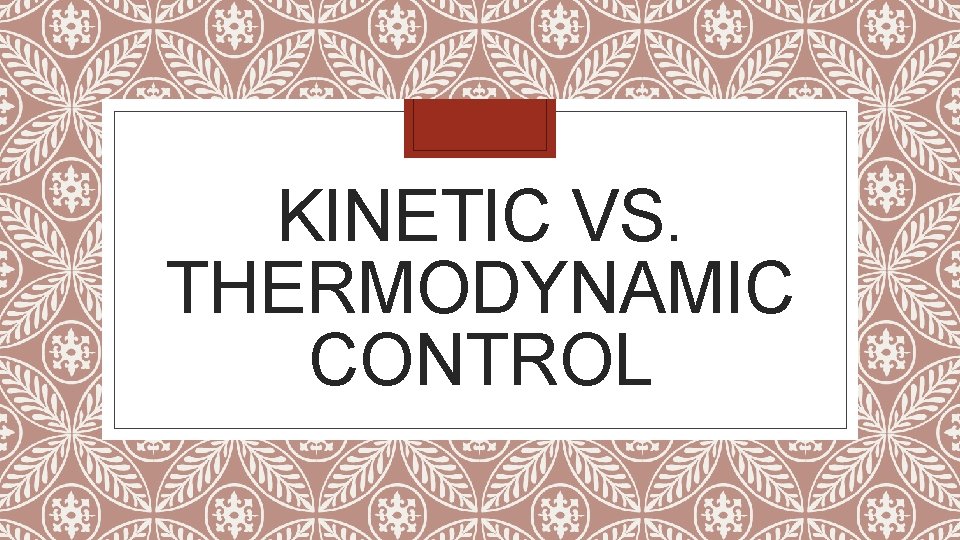
KINETIC VS. THERMODYNAMIC CONTROL
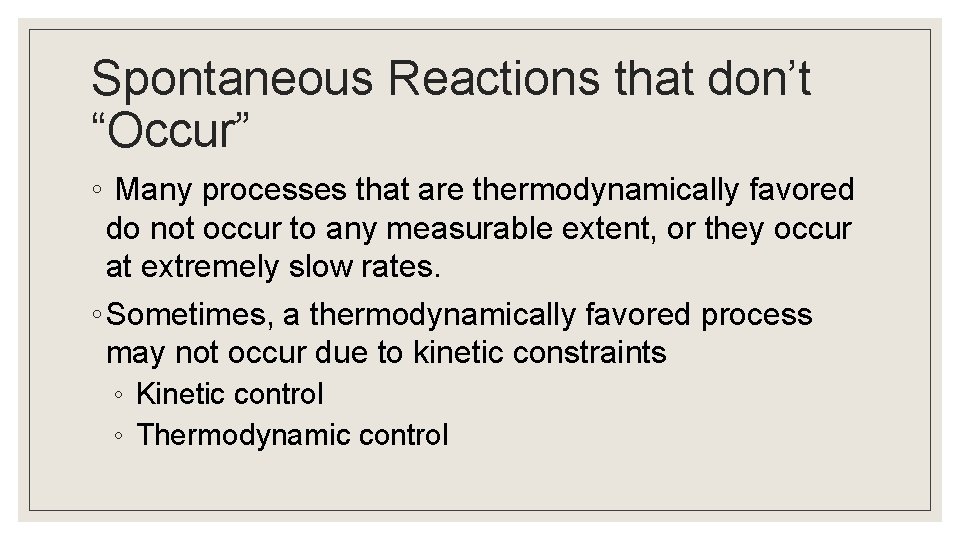
Spontaneous Reactions that don’t “Occur” ◦ Many processes that are thermodynamically favored do not occur to any measurable extent, or they occur at extremely slow rates. ◦ Sometimes, a thermodynamically favored process may not occur due to kinetic constraints ◦ Kinetic control ◦ Thermodynamic control
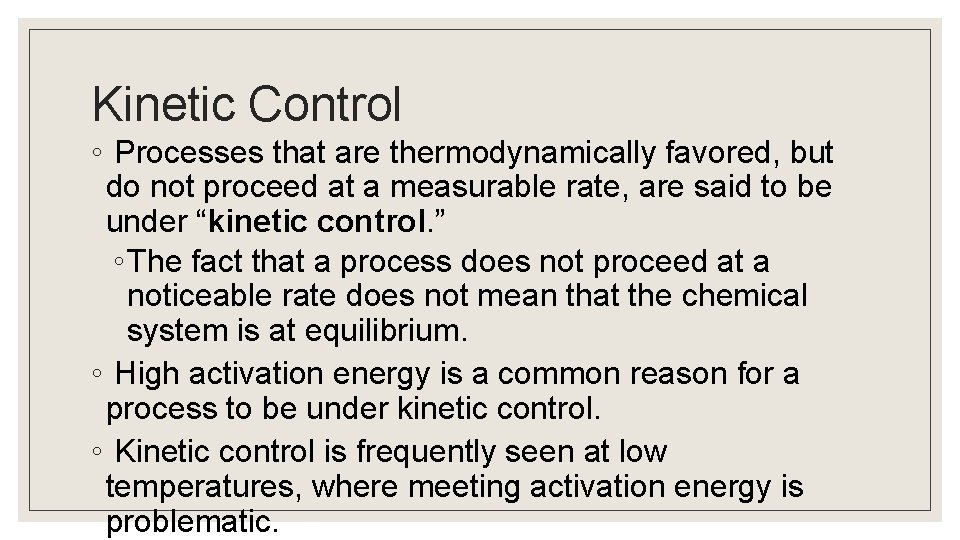
Kinetic Control ◦ Processes that are thermodynamically favored, but do not proceed at a measurable rate, are said to be under “kinetic control. ” ◦ The fact that a process does not proceed at a noticeable rate does not mean that the chemical system is at equilibrium. ◦ High activation energy is a common reason for a process to be under kinetic control. ◦ Kinetic control is frequently seen at low temperatures, where meeting activation energy is problematic.
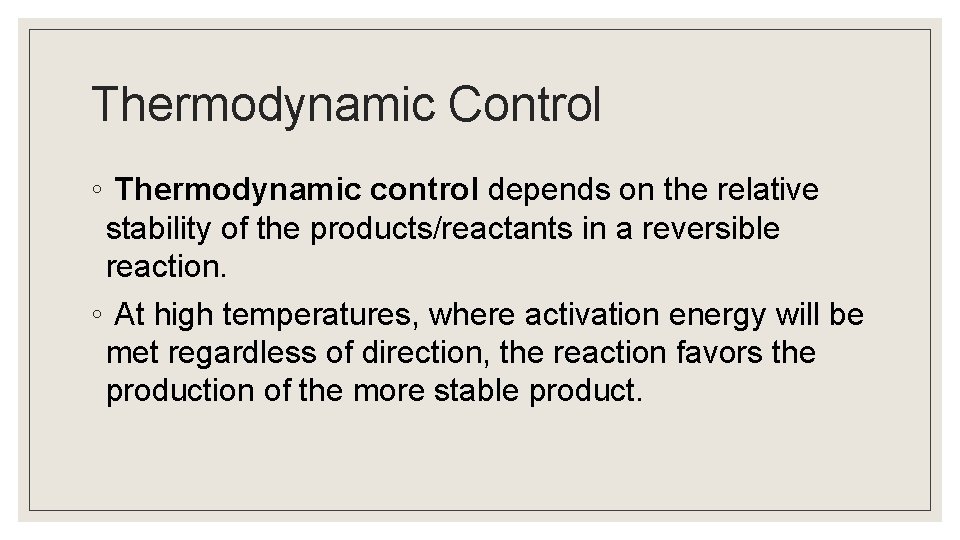
Thermodynamic Control ◦ Thermodynamic control depends on the relative stability of the products/reactants in a reversible reaction. ◦ At high temperatures, where activation energy will be met regardless of direction, the reaction favors the production of the more stable product.
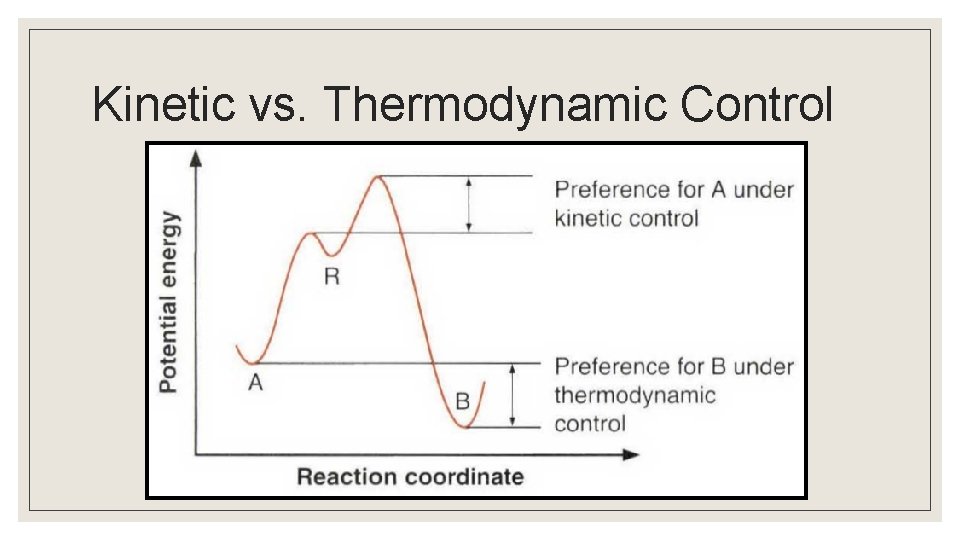
Kinetic vs. Thermodynamic Control
Chemistry semester 2 review unit 12 thermochemistry
Q=mc∆t
Endothermic equation
Lesson 10 thermodynamics unit review
Unit 10, unit 10 review tests, unit 10 general test
Gibbs free energy unit
Chapter 17 thermochemistry practice problems answers
Thermochemistry is the study of
Thermochemistry is study of: *
Balanced thermochemical equation
Introduction to thermochemistry
Zero point energy correction gaussian
Thermochemistry equations
Thermochemistry clipart
Thermochemistry is the study of...
Thermochemistry equations
Symbolic border one pager
Thermochemical equation examples
Kirchhoff's law enthalpy example
Chapter 17 thermochemistry
Thermochemistry equations
Thermochemistry cartoon
Ap chemistry thermodynamics
Unit 7 ap chemistry
Thermochemistry is study of
General chemistry thermochemistry
Bab 5
Study of energy and its transformations
Chapter 17 thermochemistry answer key
Termokimia adalah cabang ilmu yang mempelajari
What is thermochemistry
What is thermochemistry
Quiz 2: heat flow and technology
Thermochemistry concepts
Thermochemistry is study of
Energy diagram thermochemistry
Thermochemistry is concerned with the
Kinetic energy thermochemistry
Thermochemistry
A piece of metal is heated then submerged in cool water
Bomb calorimetry equation
Thermochemistry video
Thermochemistry
Thermochemistry
Thermochemistry exam review
Hess law constant heat summation
Calorimeter
Q = m x cp x delta t
Thermochemistry
Why are chemists interested in studying thermochemistry?
Chapter 6 thermochemistry
Chapter 17 thermochemistry
Thermochemistry vocabulary
Why do we study thermodynamics
Thermodynamics ppt
What is thermodynamics equilibrium
Thermodynamics property tables
Internal energy formula thermodynamics
Sssf thermodynamics
Usuf thermodynamics
Thermodynamics deals with
Tds equations
Thermodynamic probability
Zeroth law of thermo
Third law of thermodynamics derivation
Zeroth law of thermodynamics statement
Engineering thermodynamics
First law of thermodynamics
1th law of thermodynamics
Residual properties in thermodynamics
Laws of thermodynamics simple
Statistical thermodynamics in chemistry
Maxwell relation
Reversible and irreversible process
Work done in isentropic process
Thermodynamics 1 formula sheet
Equilibrium constant in thermodynamics
Refrigerant cop
Entropy thermodynamics
Properties of pure substances thermodynamics
Thermodynamics study guide
Maxwell's thermodynamic relations
State second law of thermodynamics
First law of thermodynamics experiment
Heat capacity
What is brayton cycle in thermodynamics
Chapter 6
Chemical engineering thermodynamics 8th solution chapter 3
Thermodynamics for chemical engineering
What is internal energy
Difference between internal energy and enthalpy
Raoult's law and dalton's law
Thermodynamics first law
First law of td
Steady flow process thermodynamics
What is entropy in thermodynamics
çengel
What is brayton cycle in thermodynamics