Thermodynamics Unit 6 6 1 Endothermic and Exothermic
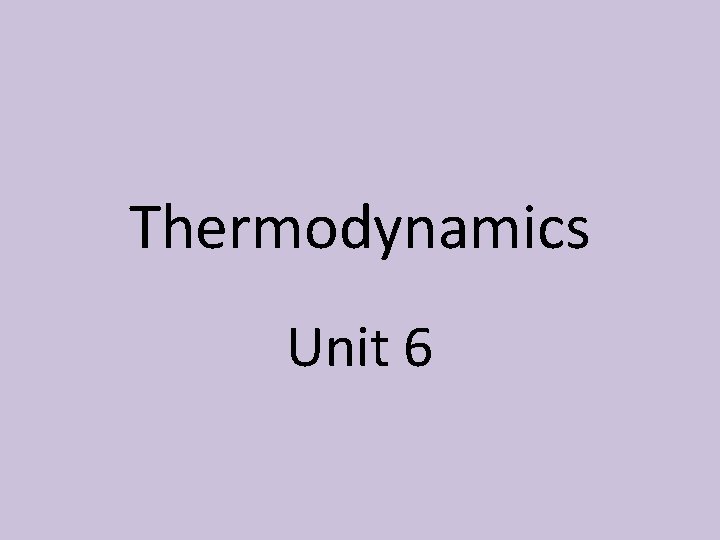
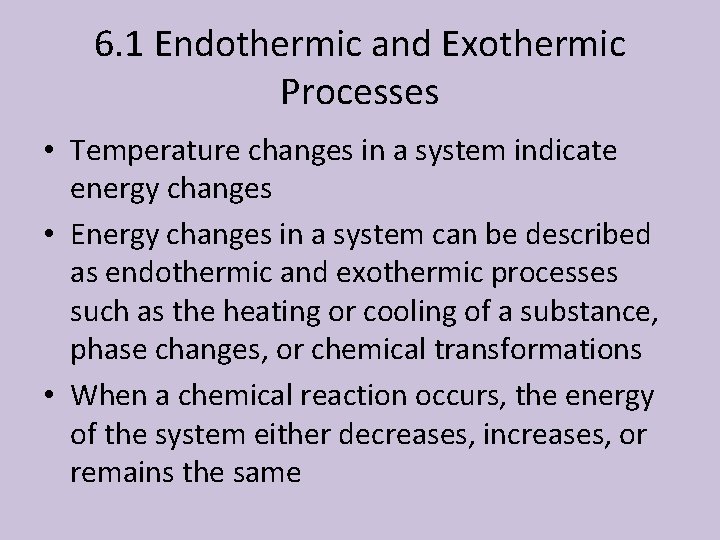
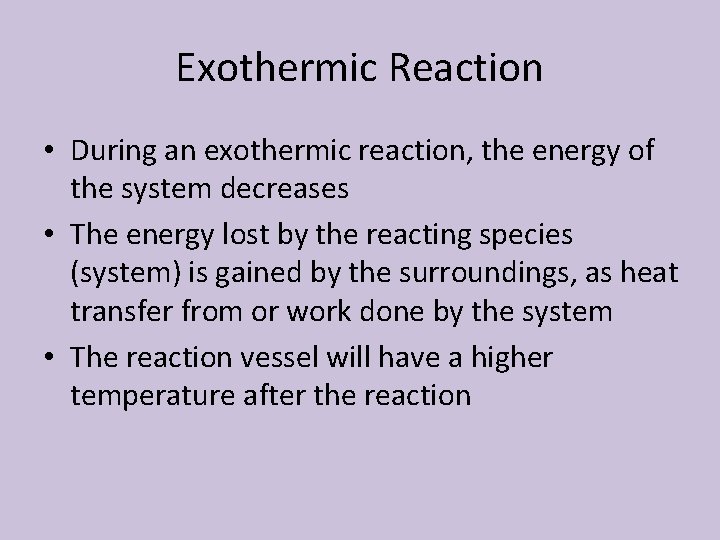
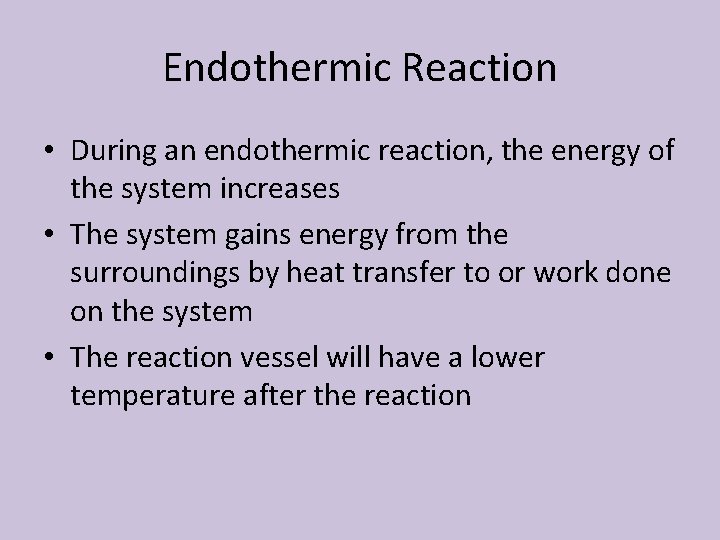
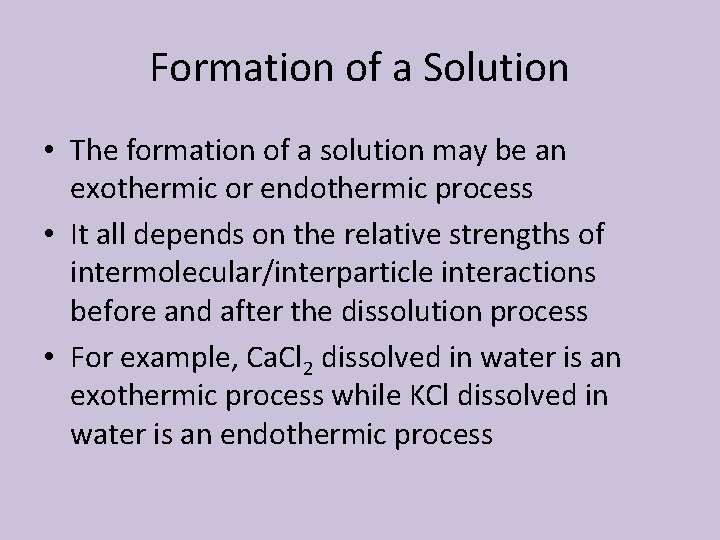
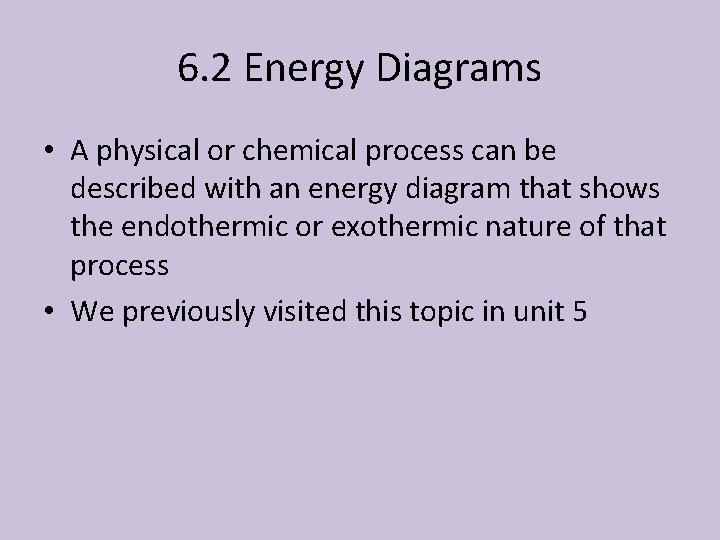
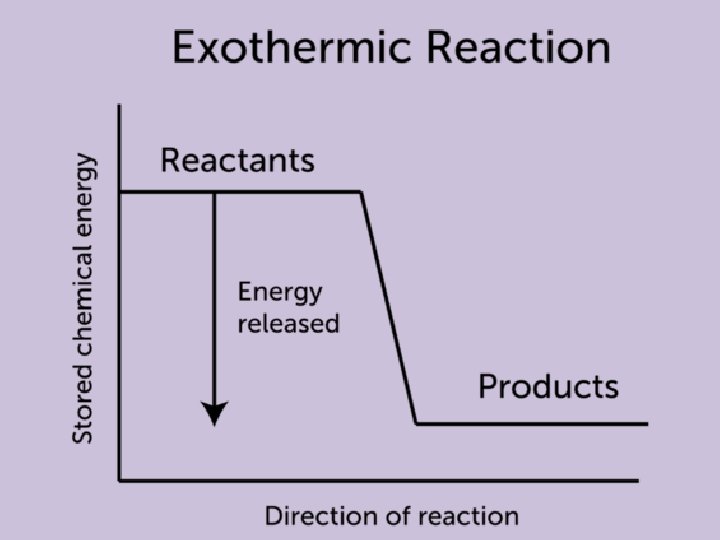
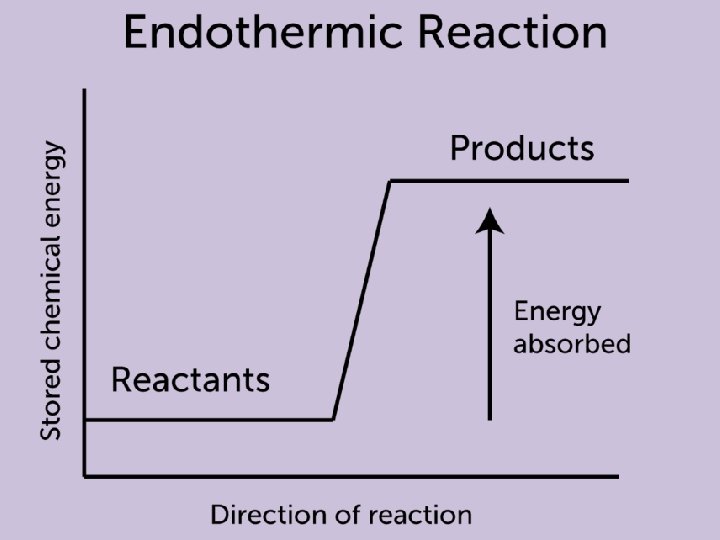
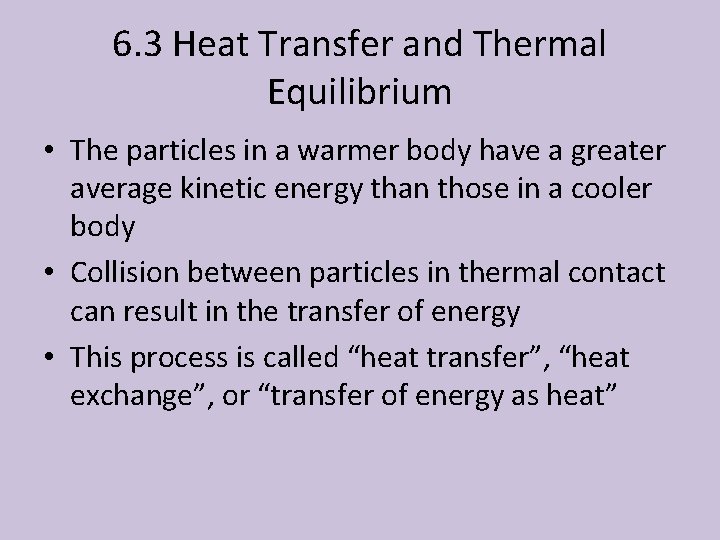
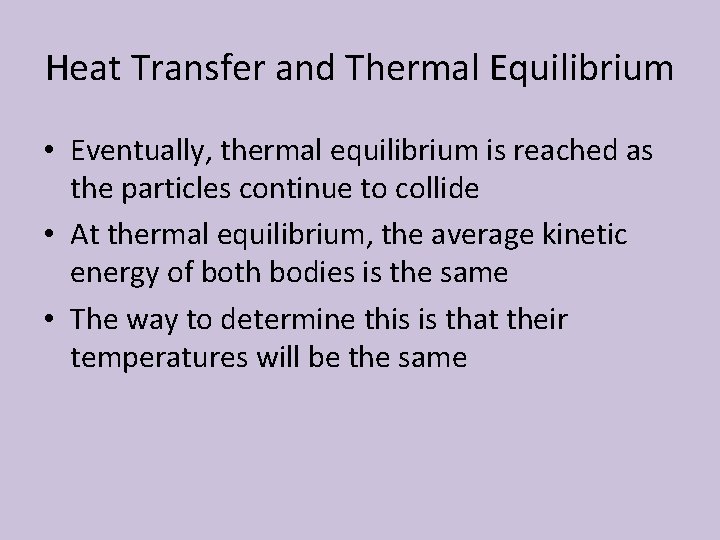
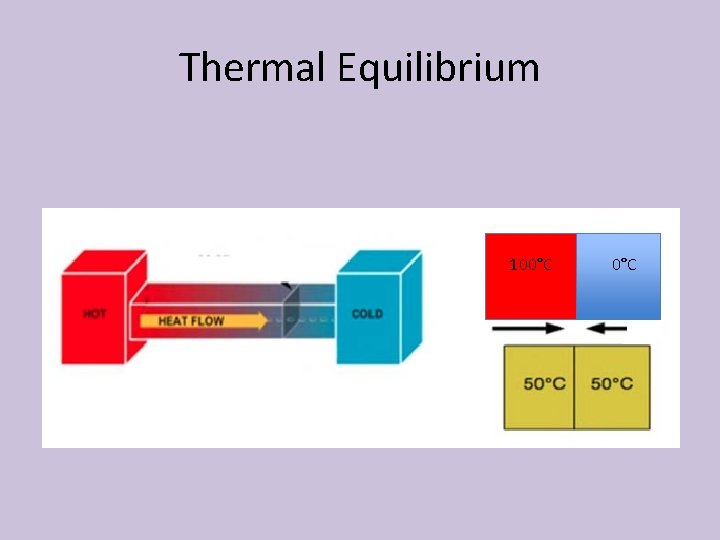
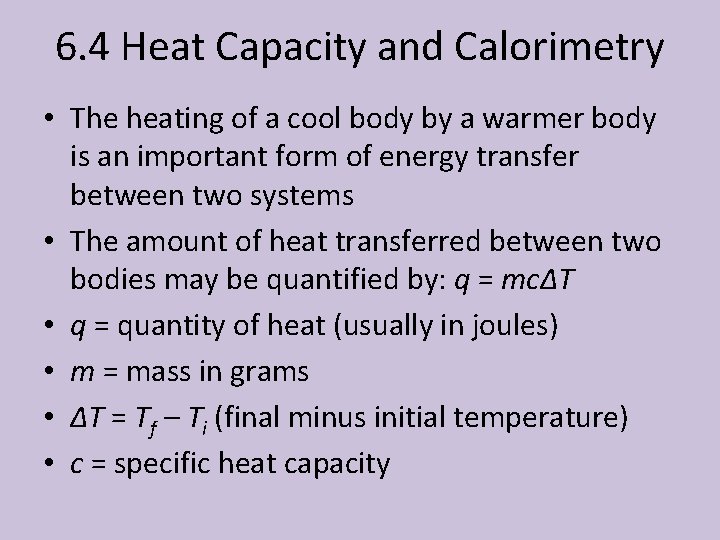
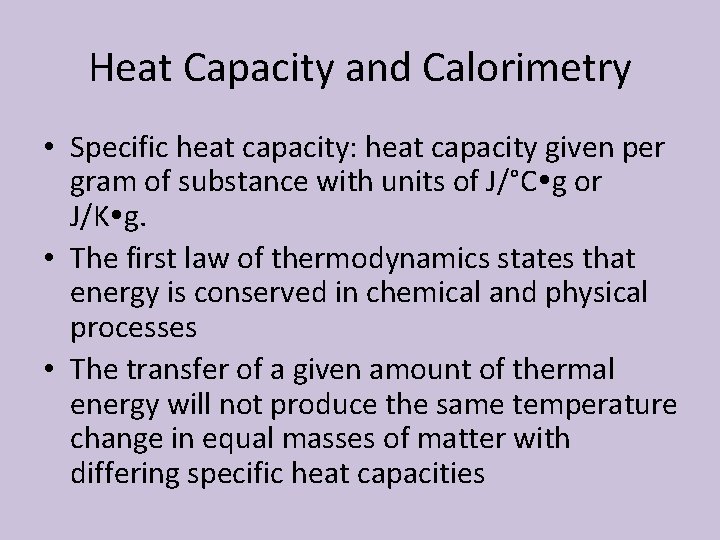
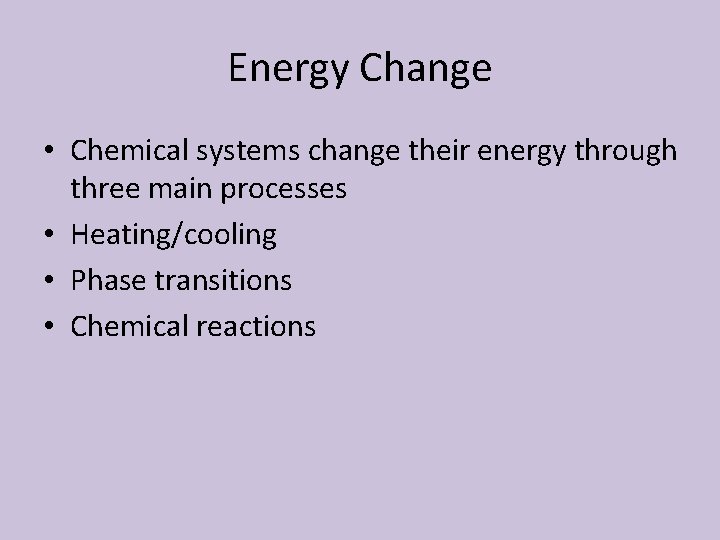
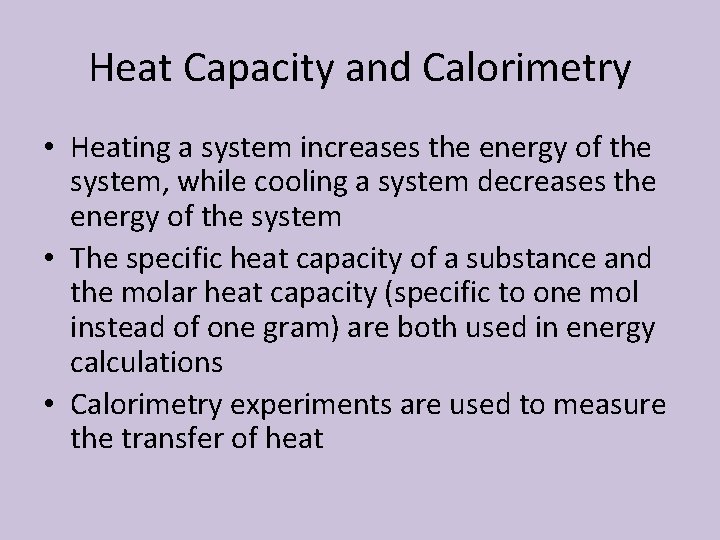
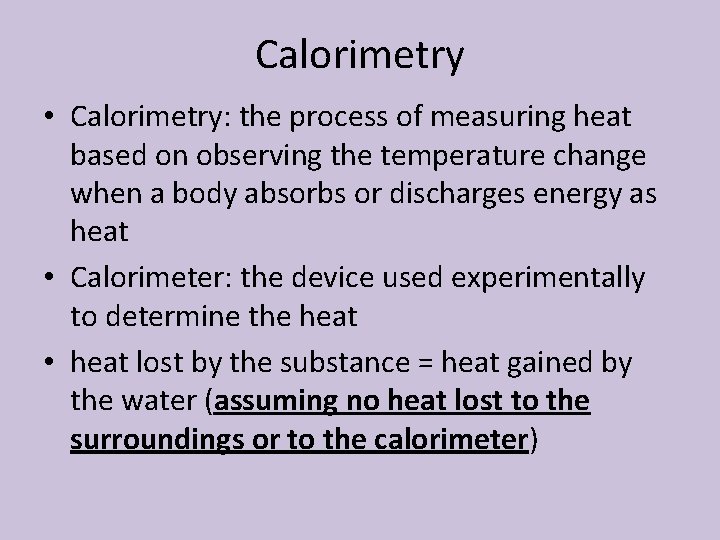
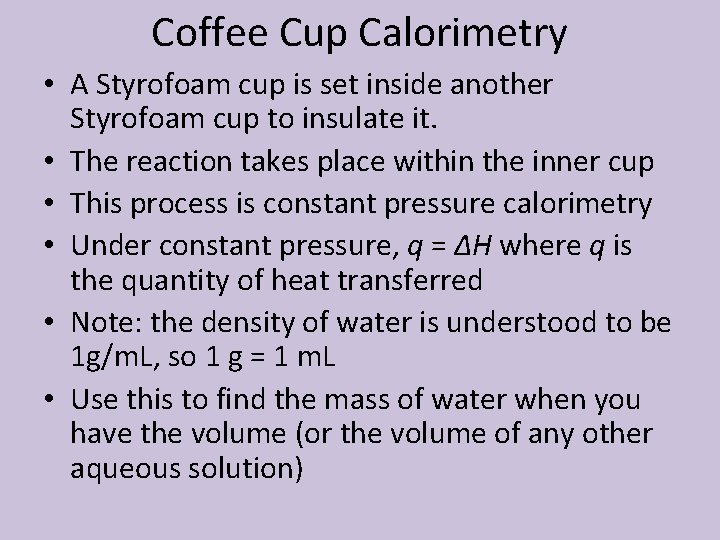
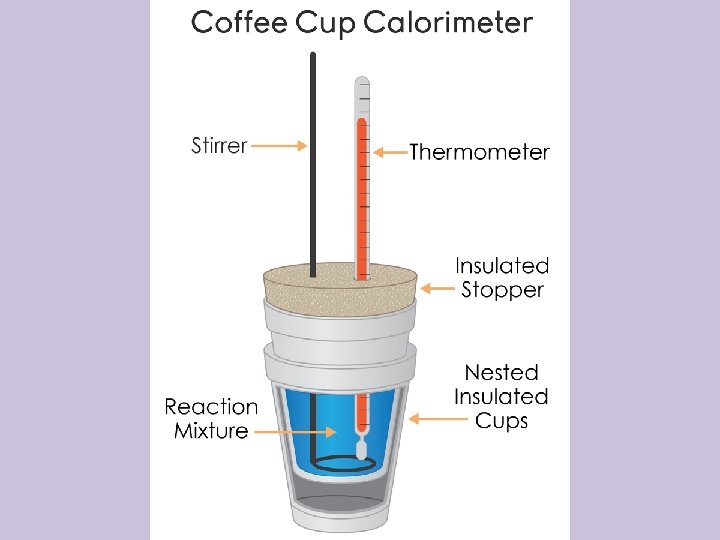
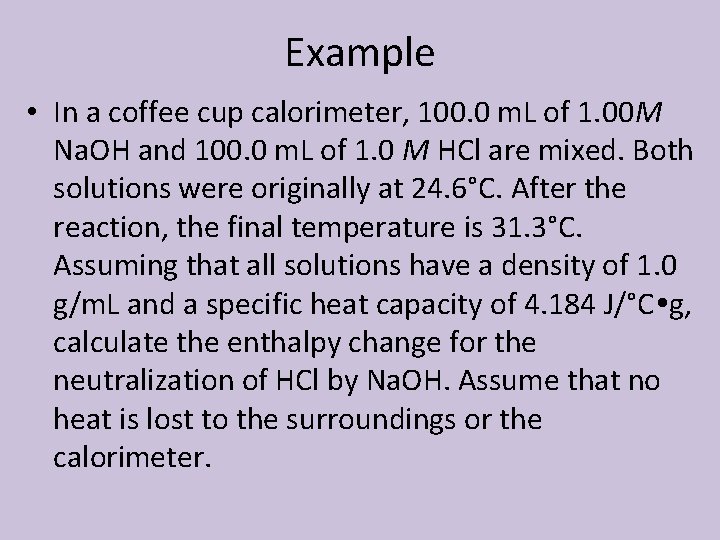
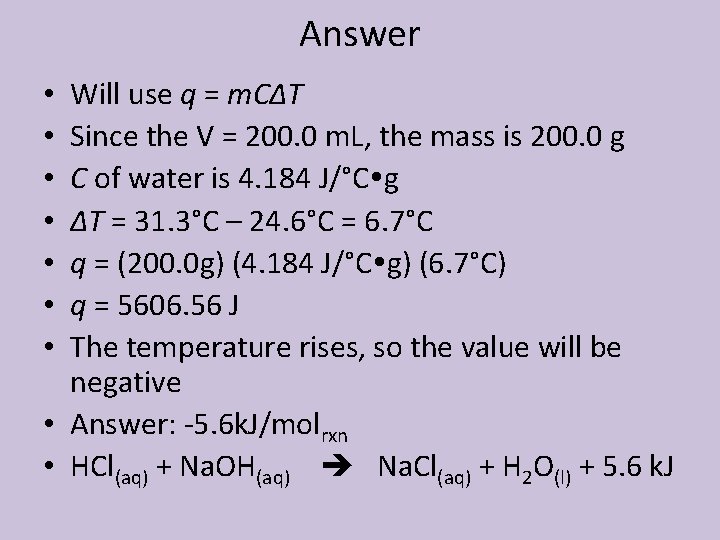
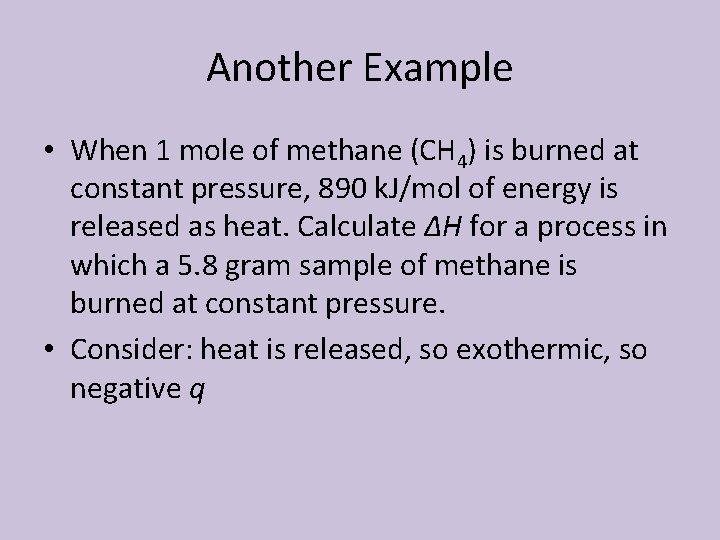
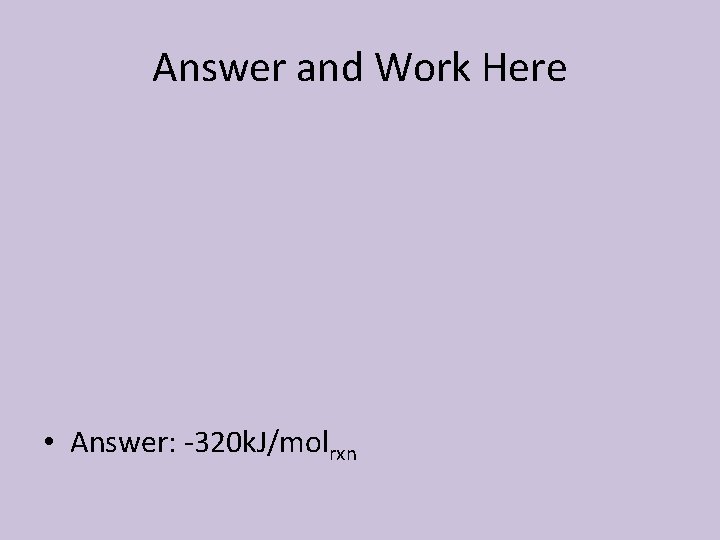
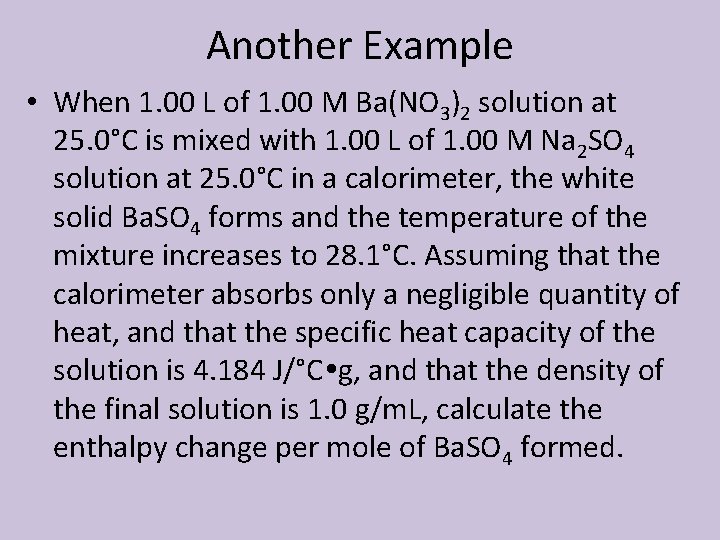
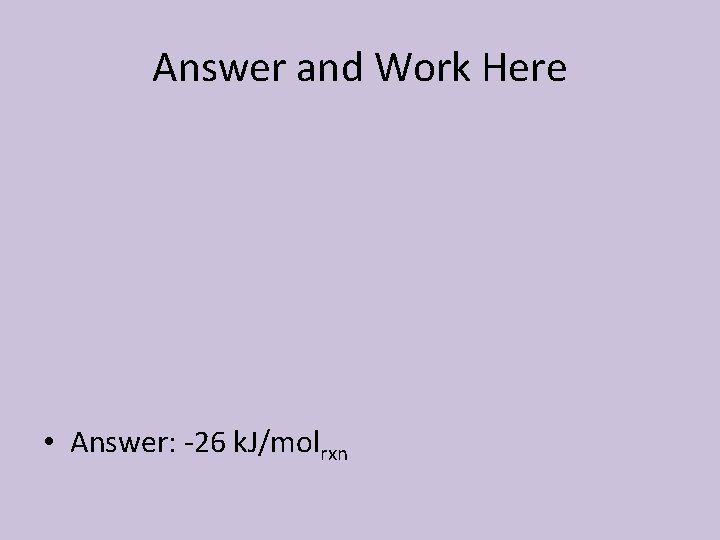
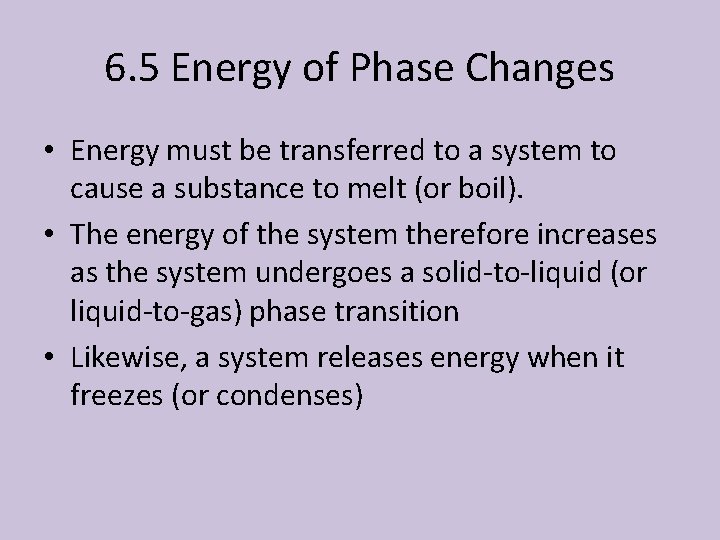
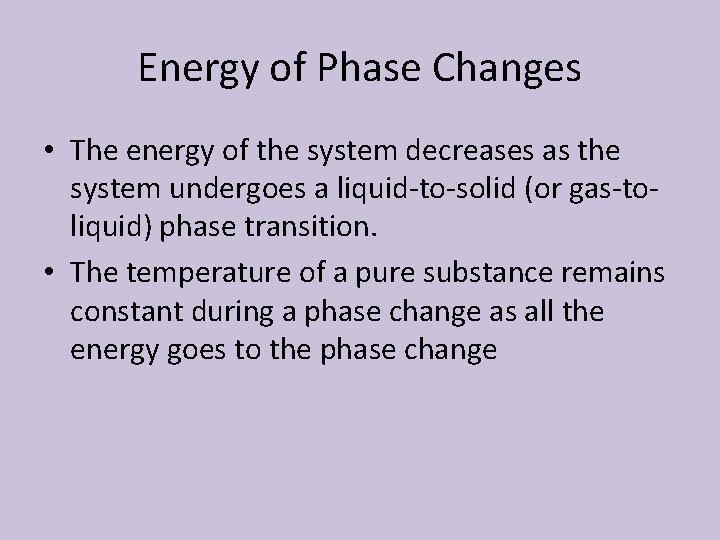
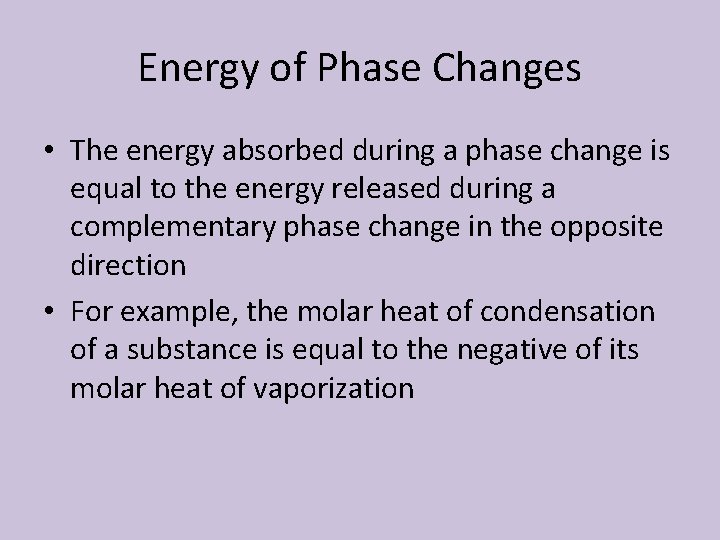
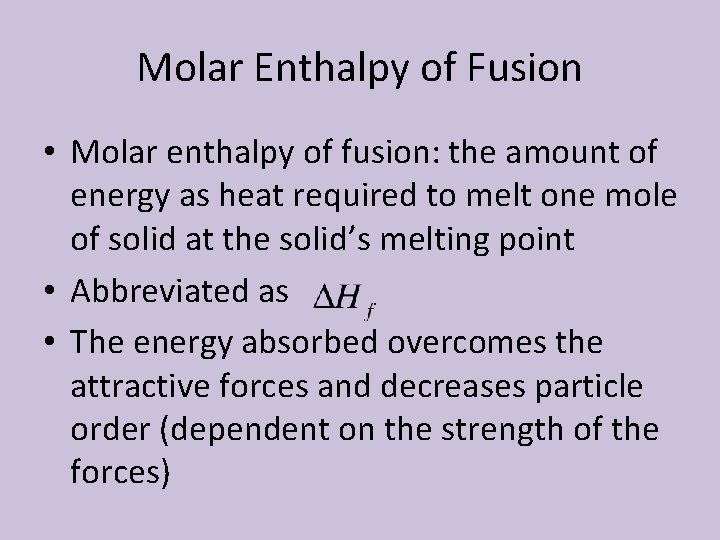
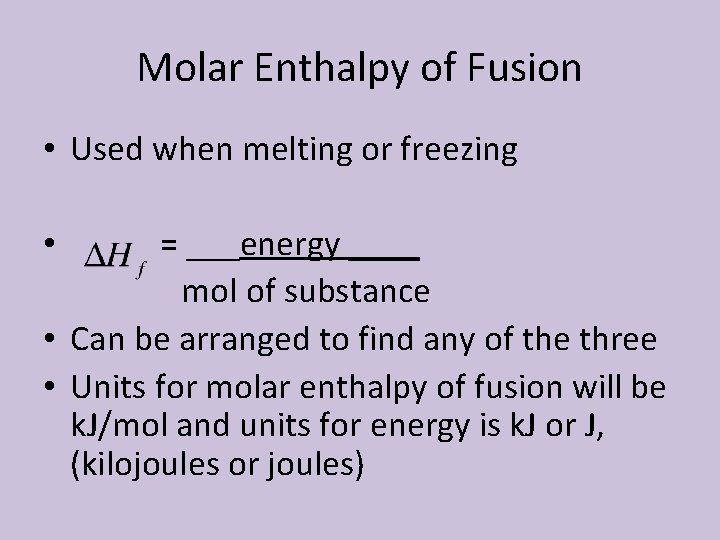
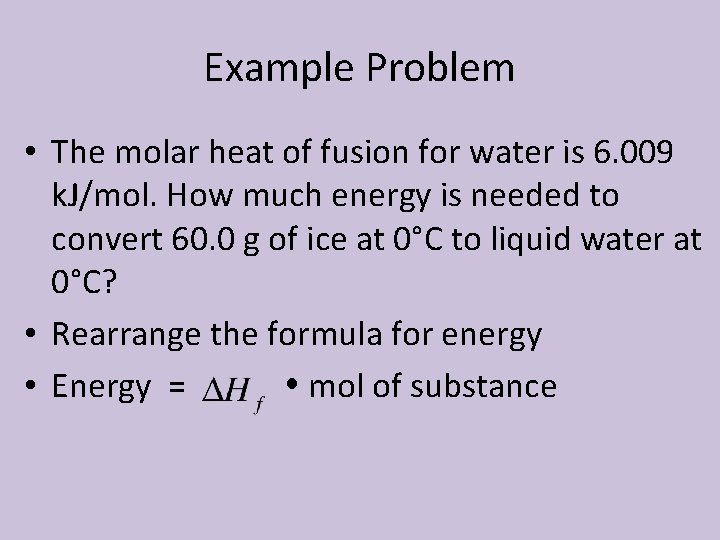
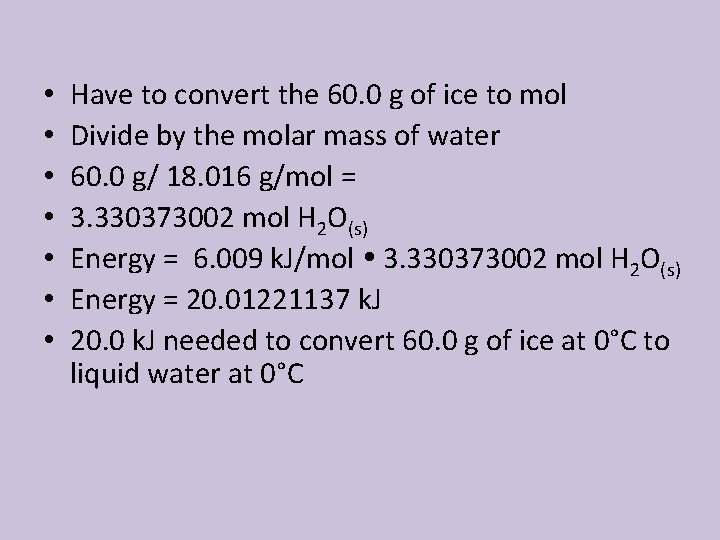
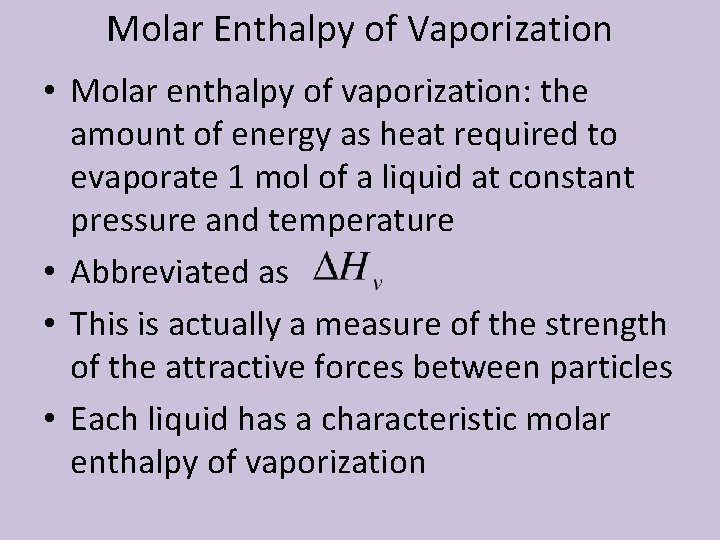
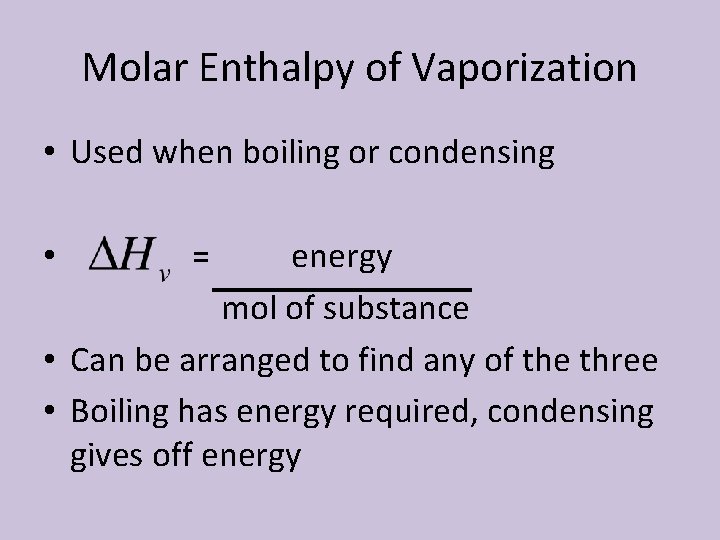
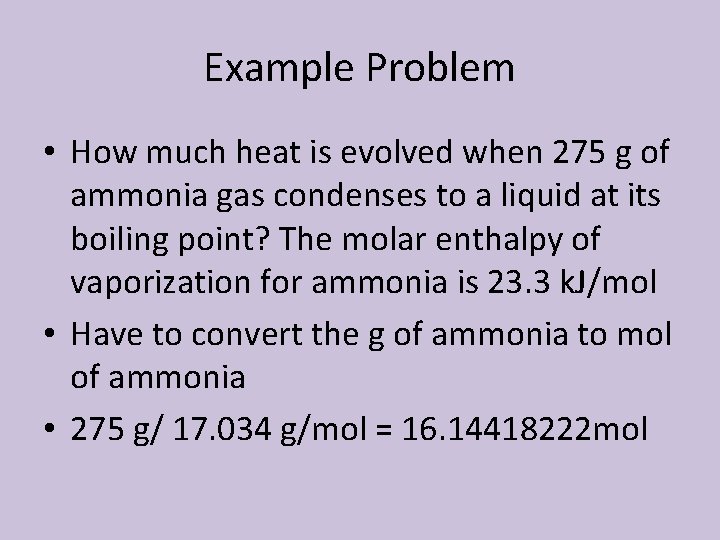
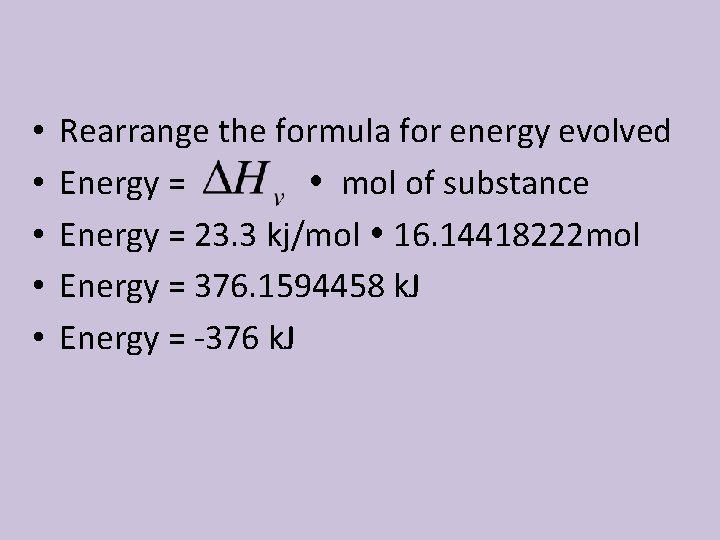
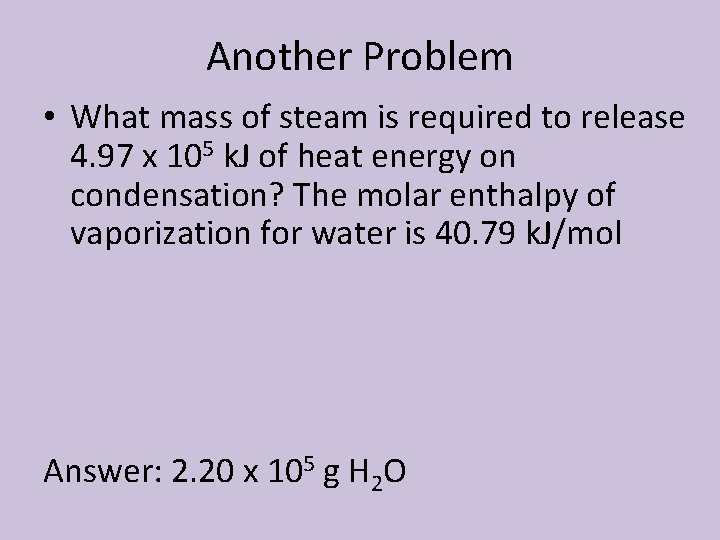
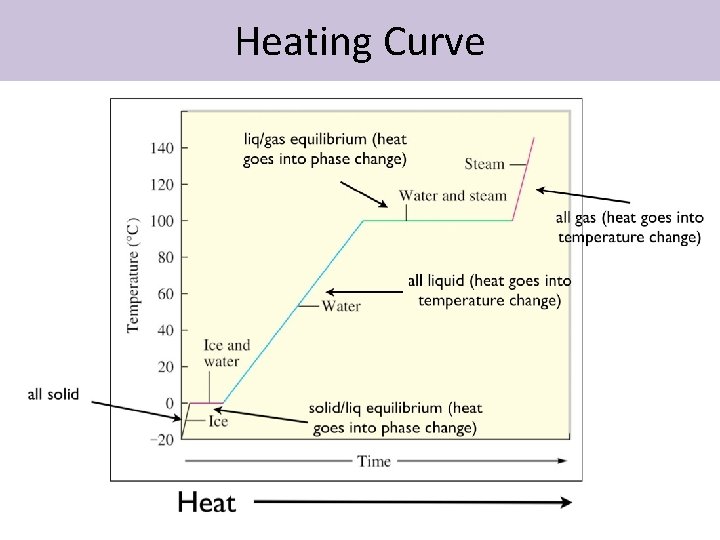
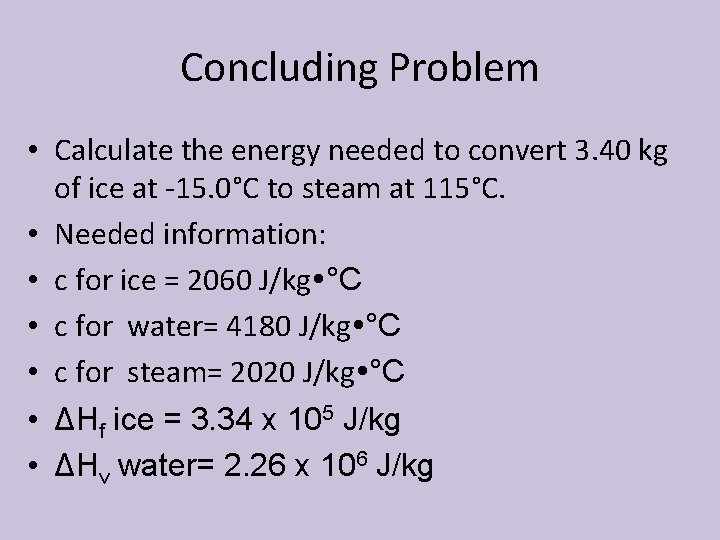
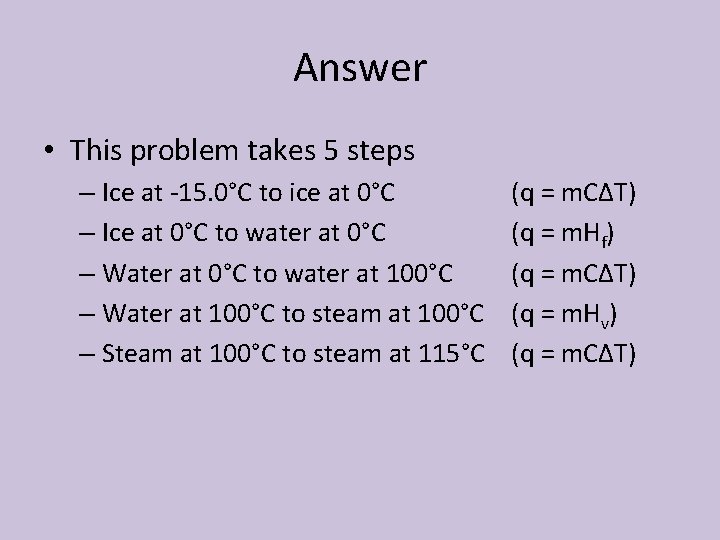
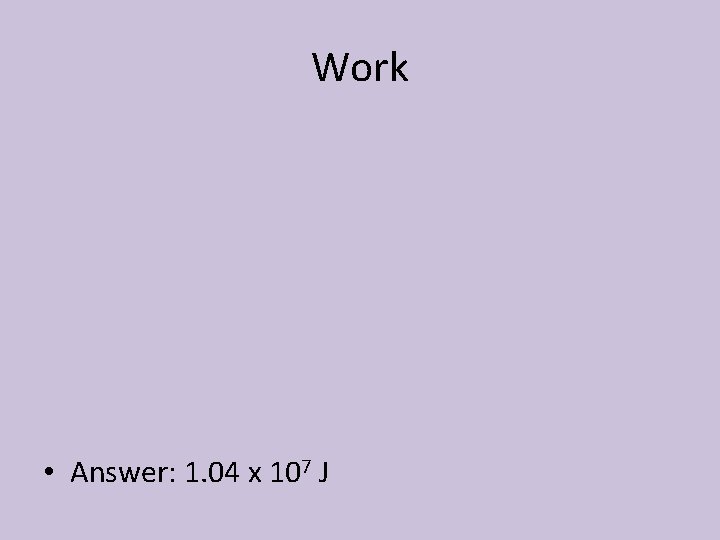
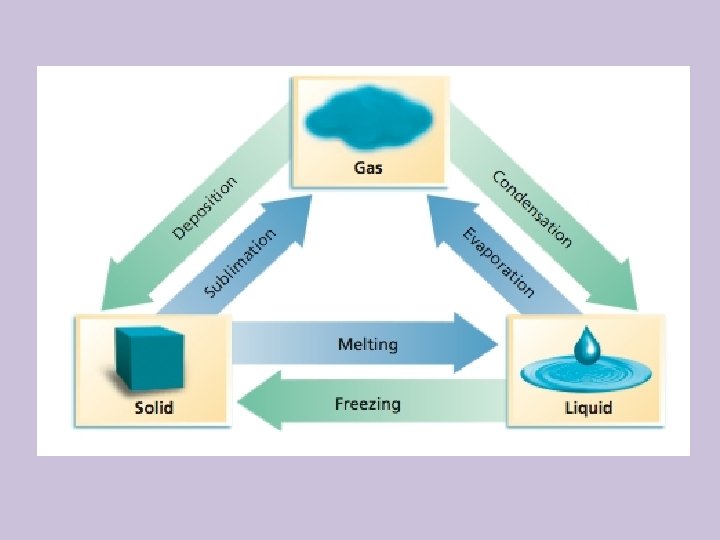
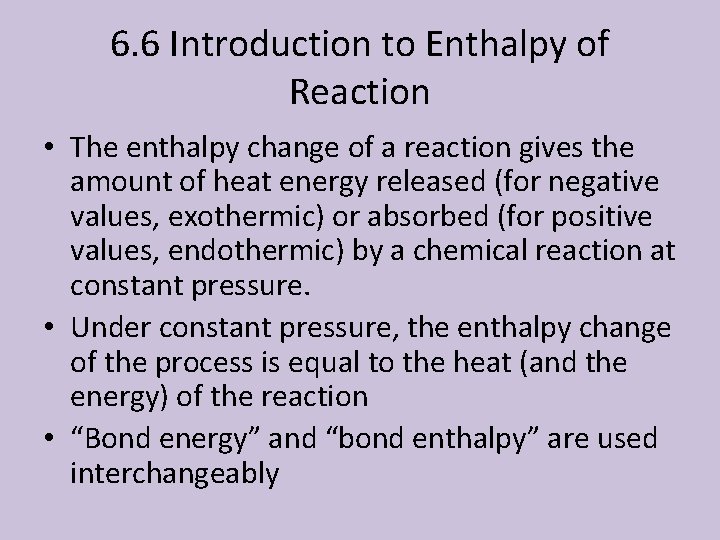
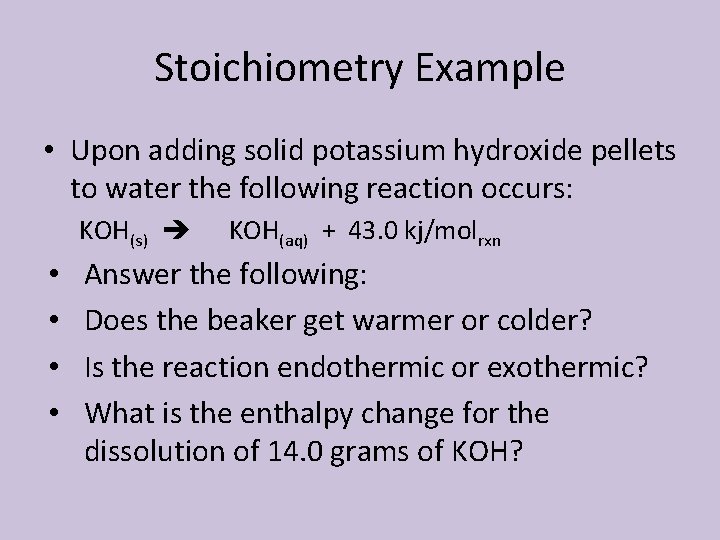
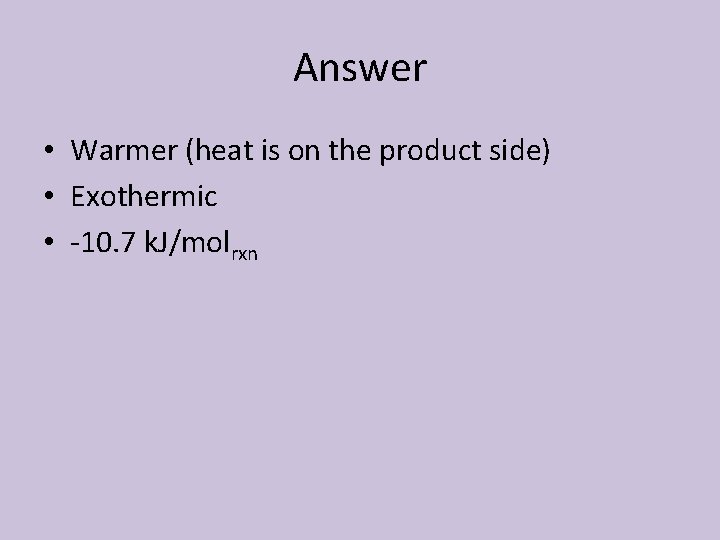
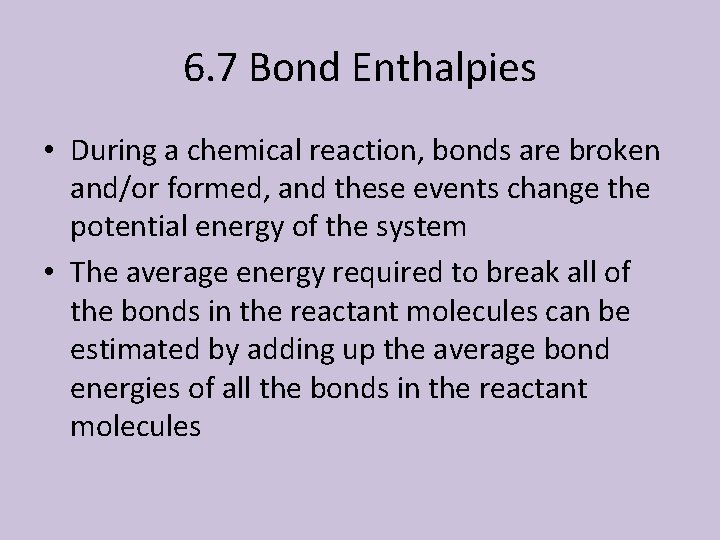
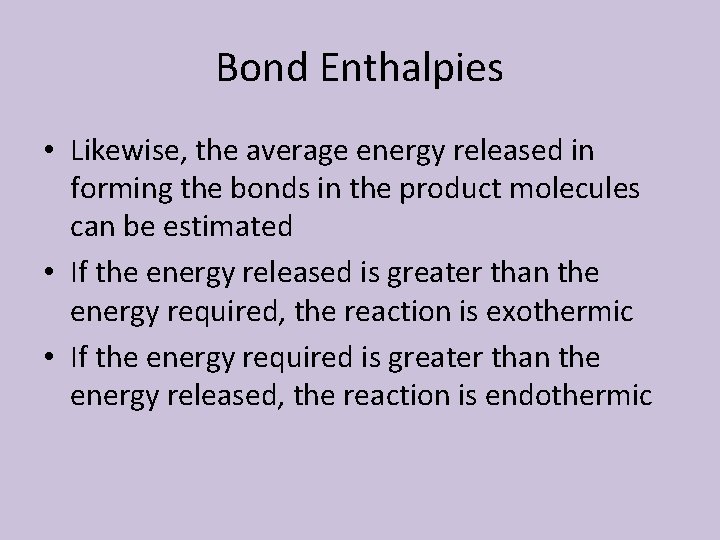
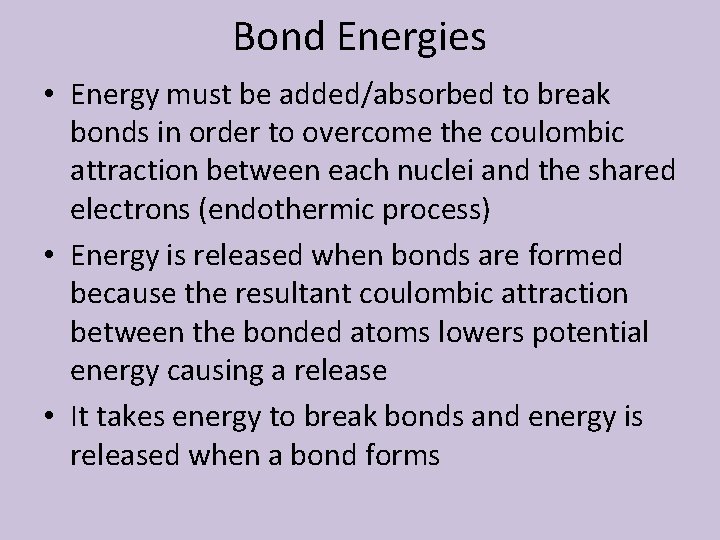
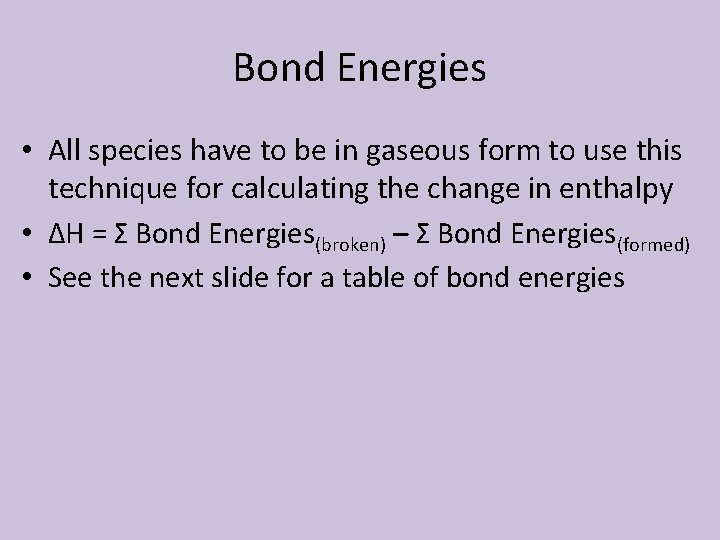
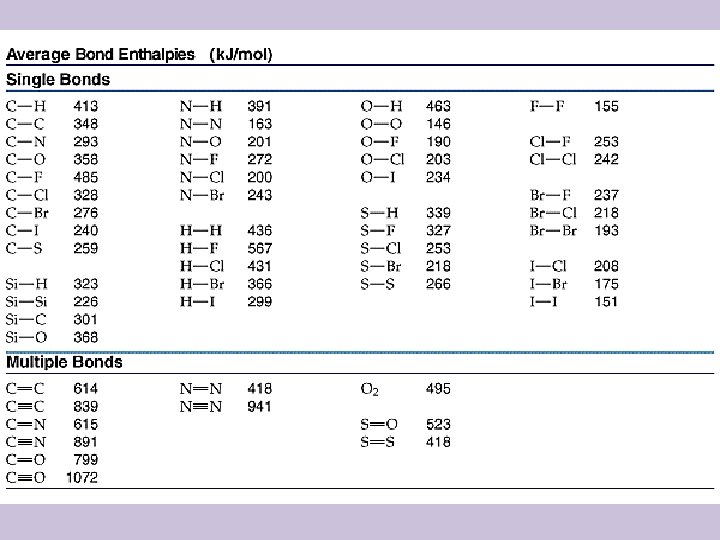
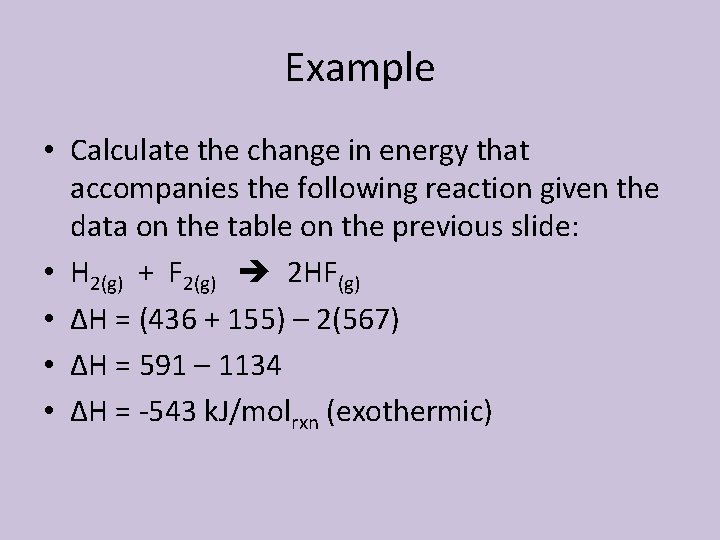
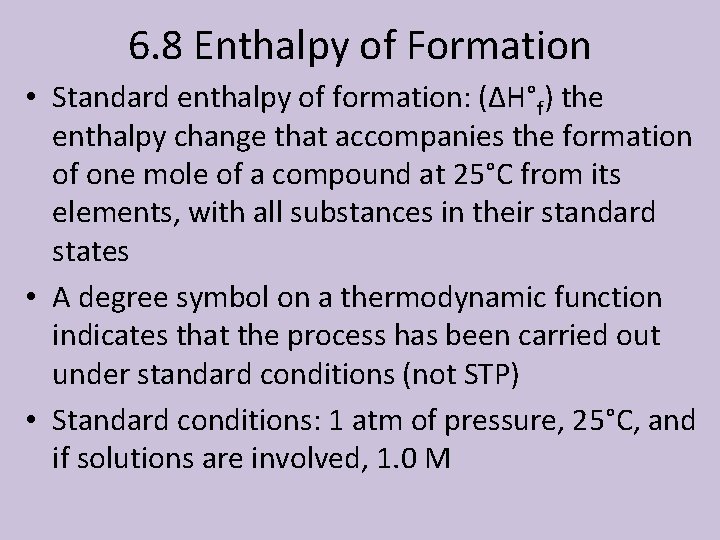
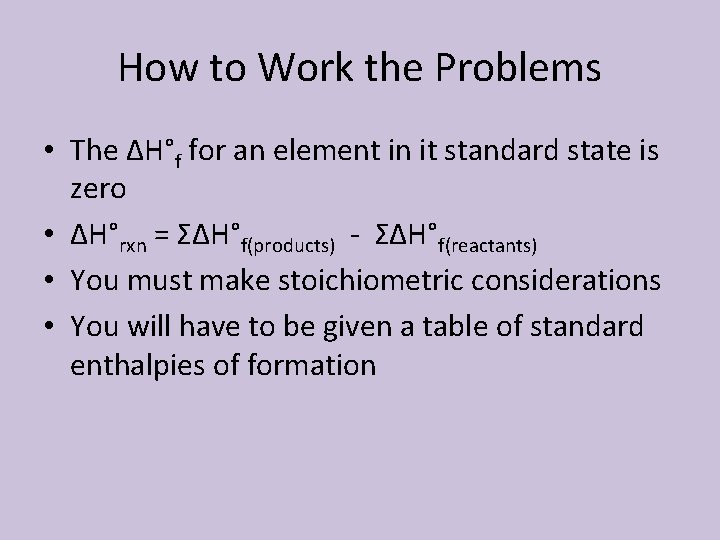
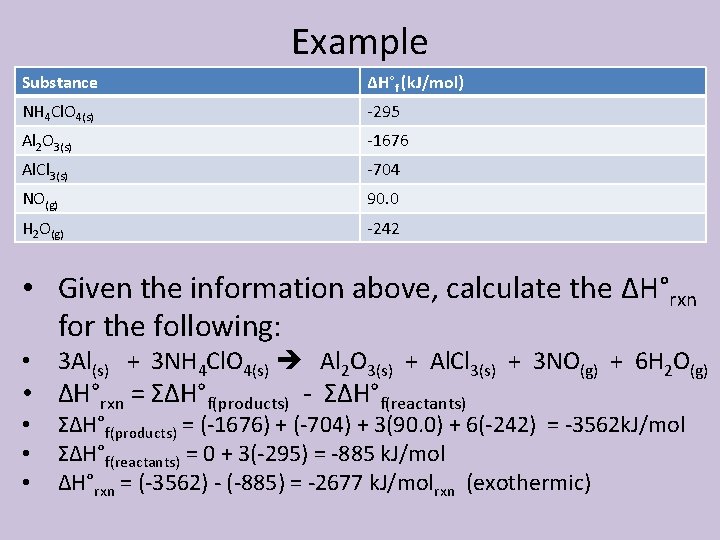
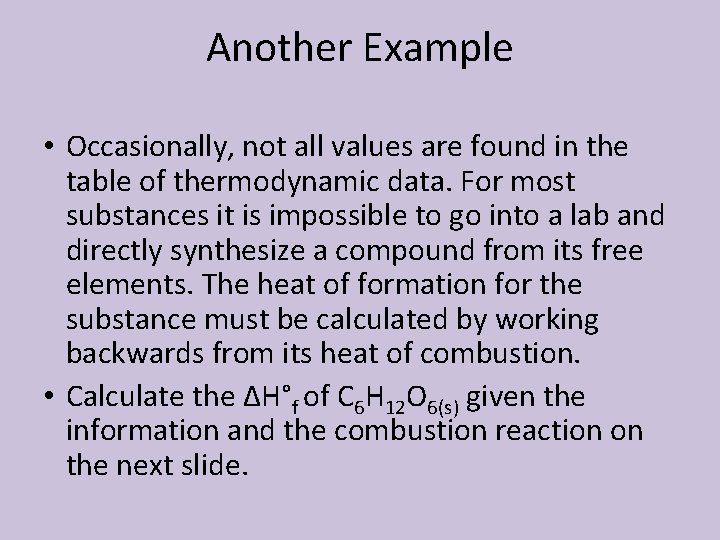
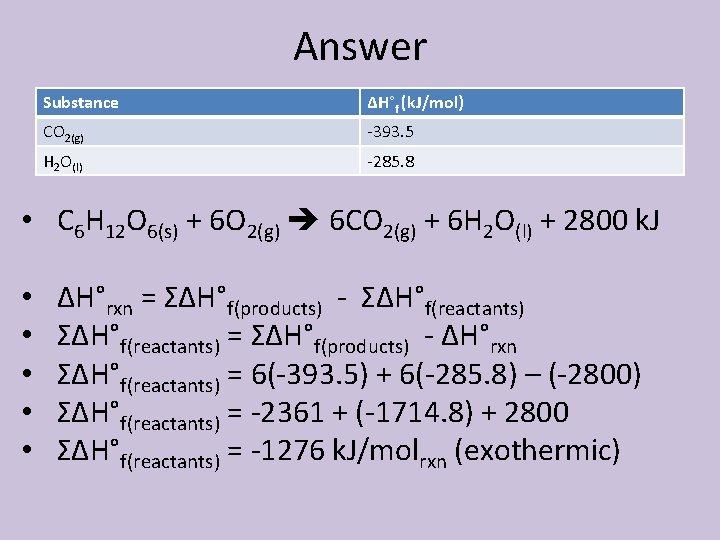
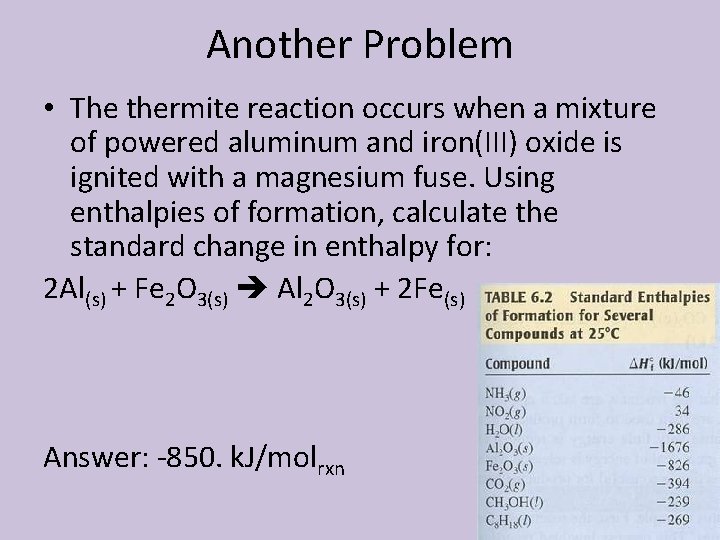
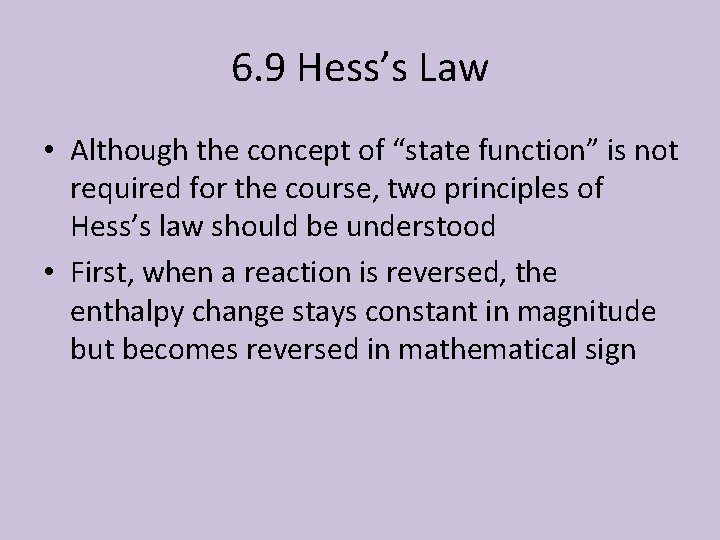
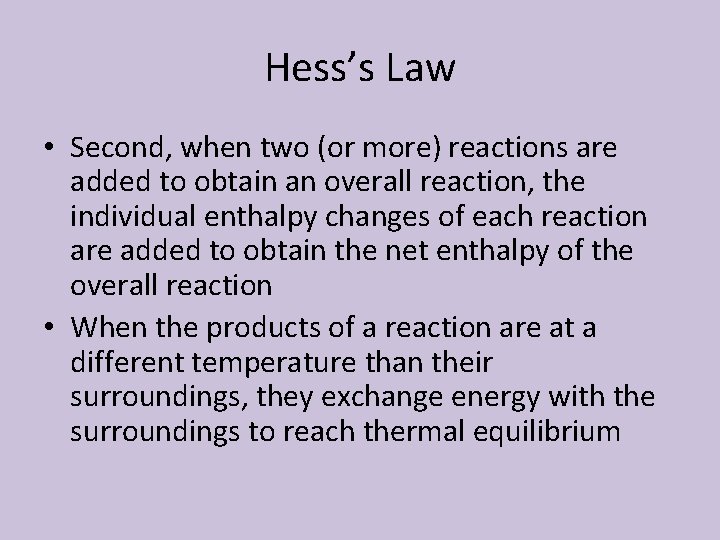
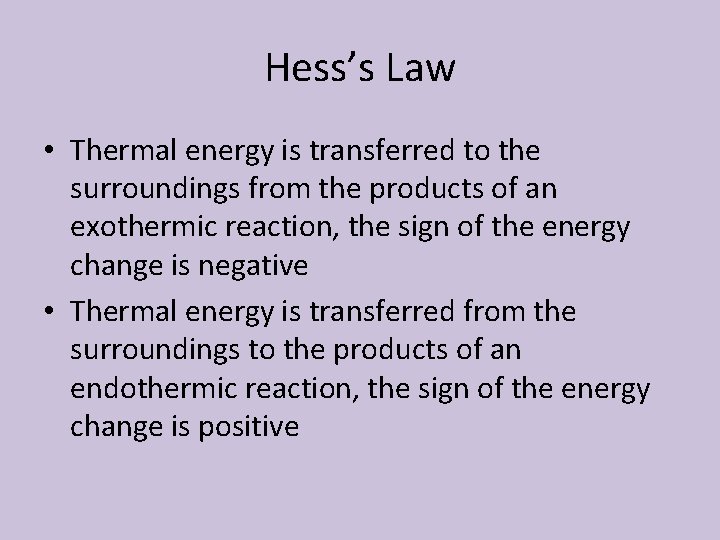
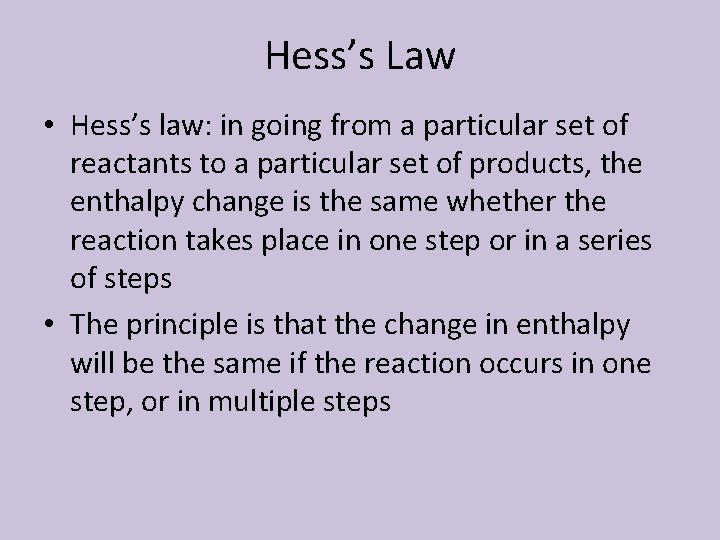
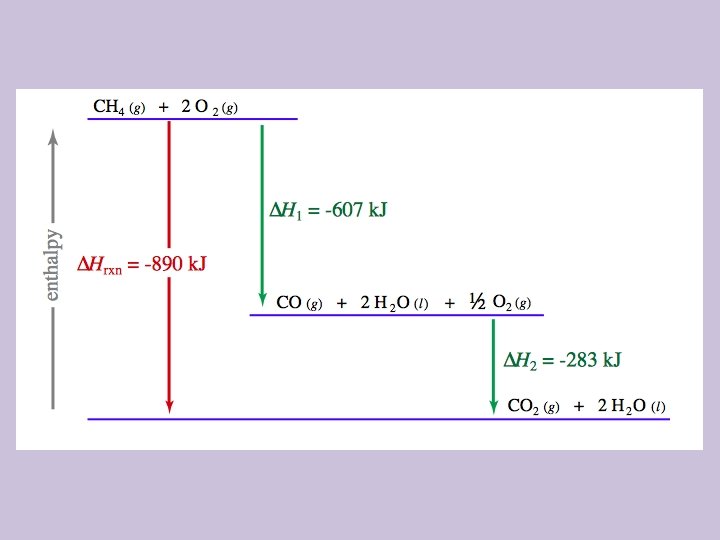
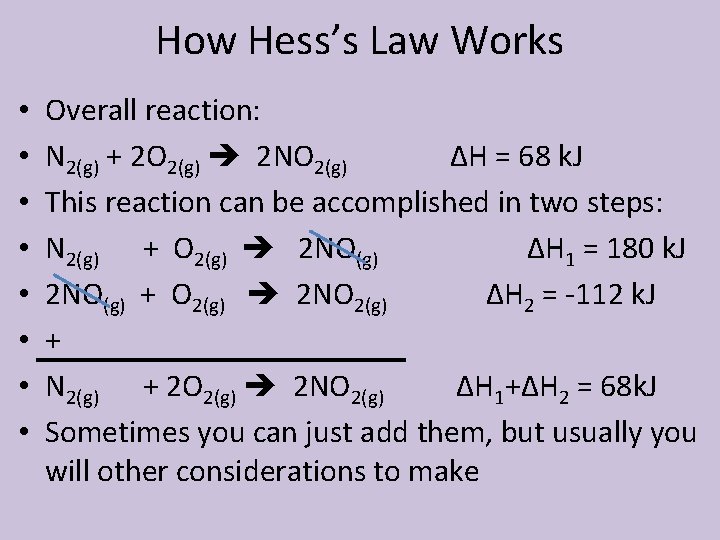
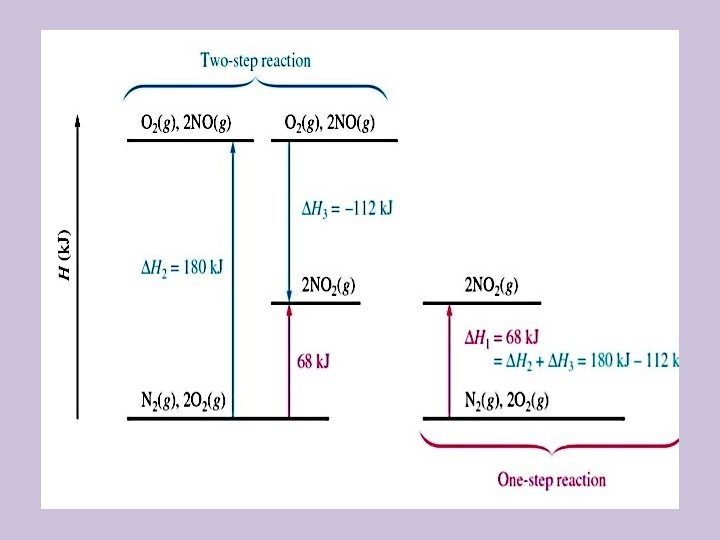
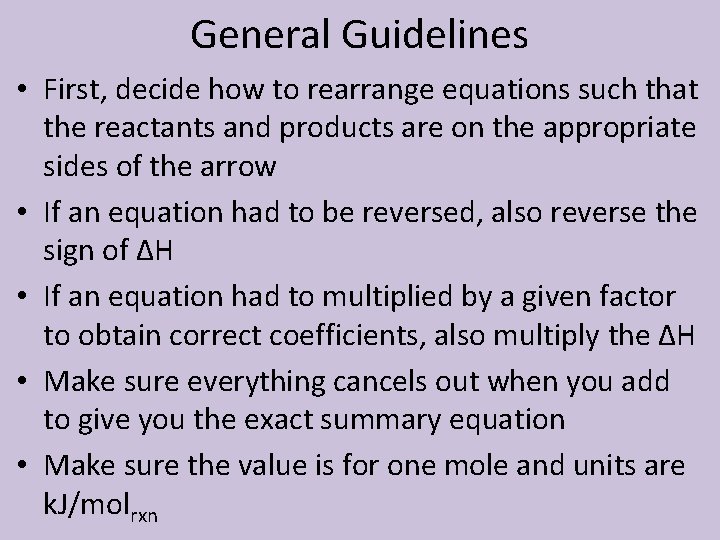
- Slides: 64
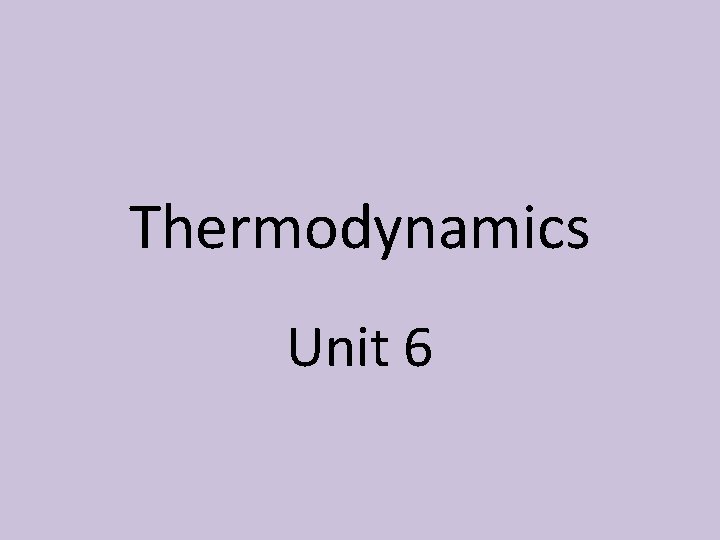
Thermodynamics Unit 6
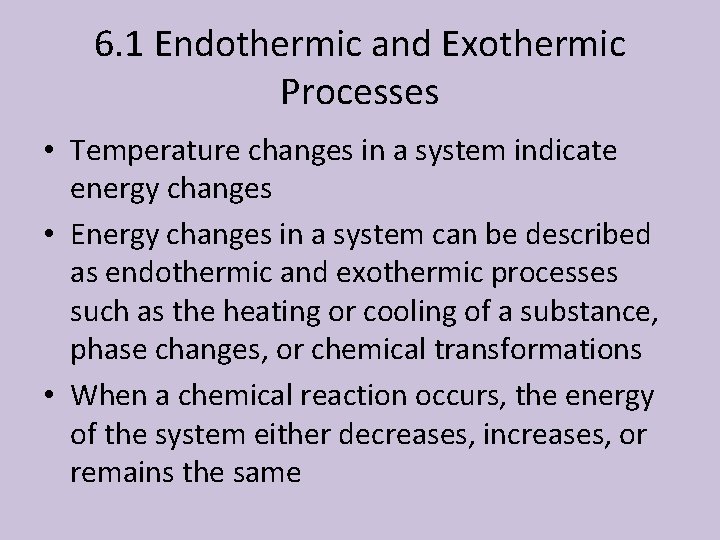
6. 1 Endothermic and Exothermic Processes • Temperature changes in a system indicate energy changes • Energy changes in a system can be described as endothermic and exothermic processes such as the heating or cooling of a substance, phase changes, or chemical transformations • When a chemical reaction occurs, the energy of the system either decreases, increases, or remains the same
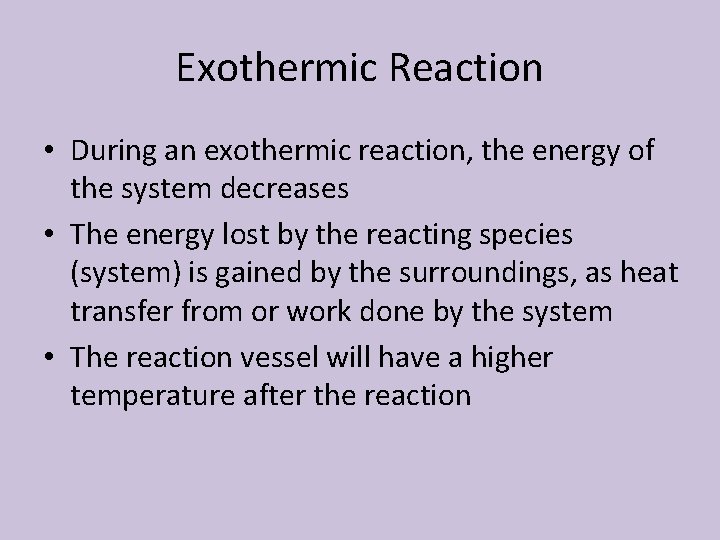
Exothermic Reaction • During an exothermic reaction, the energy of the system decreases • The energy lost by the reacting species (system) is gained by the surroundings, as heat transfer from or work done by the system • The reaction vessel will have a higher temperature after the reaction
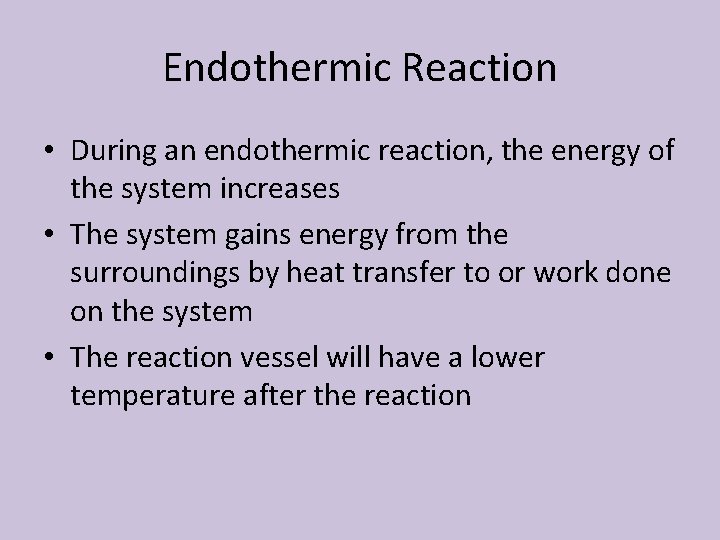
Endothermic Reaction • During an endothermic reaction, the energy of the system increases • The system gains energy from the surroundings by heat transfer to or work done on the system • The reaction vessel will have a lower temperature after the reaction
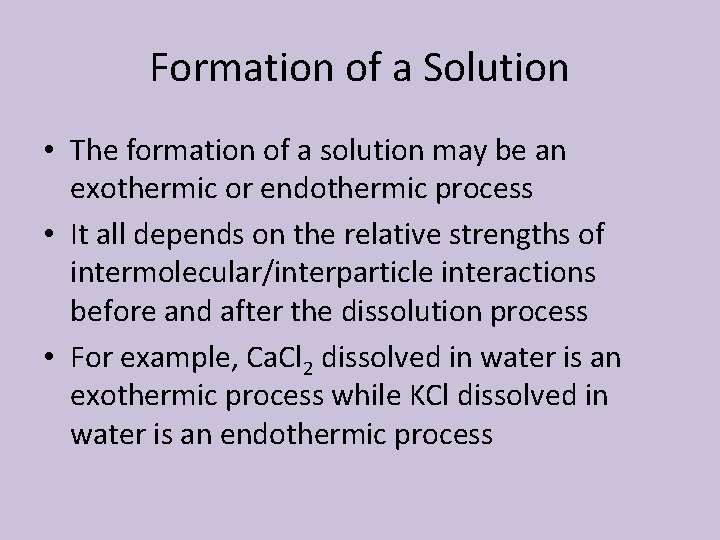
Formation of a Solution • The formation of a solution may be an exothermic or endothermic process • It all depends on the relative strengths of intermolecular/interparticle interactions before and after the dissolution process • For example, Ca. Cl 2 dissolved in water is an exothermic process while KCl dissolved in water is an endothermic process
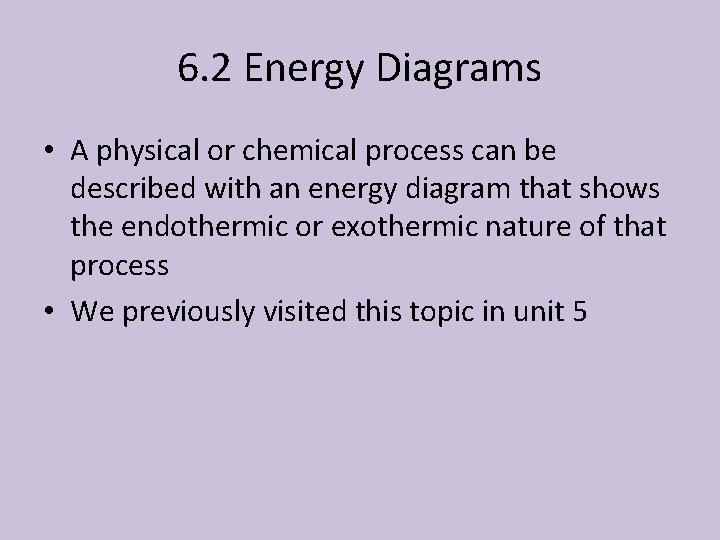
6. 2 Energy Diagrams • A physical or chemical process can be described with an energy diagram that shows the endothermic or exothermic nature of that process • We previously visited this topic in unit 5
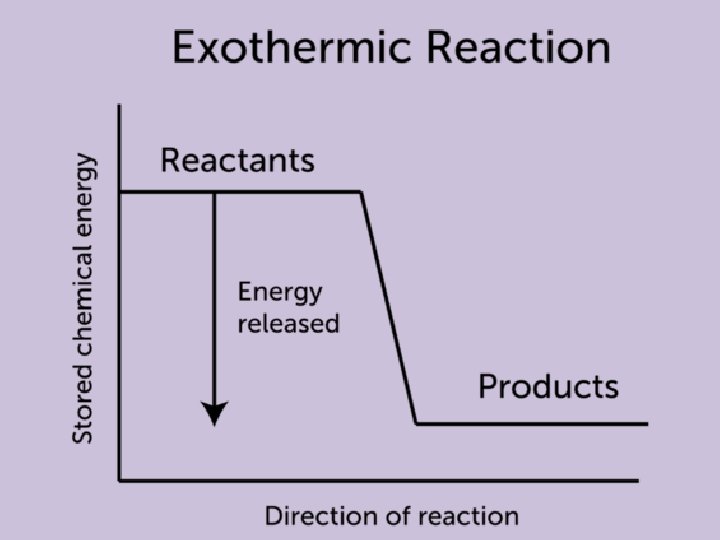
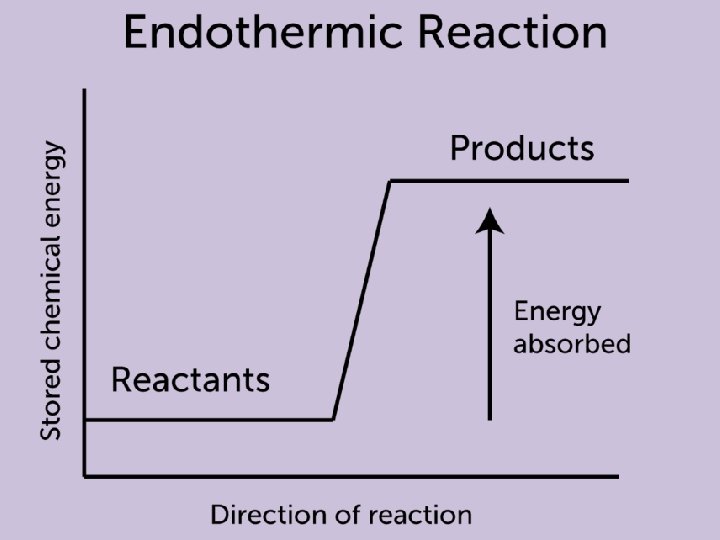
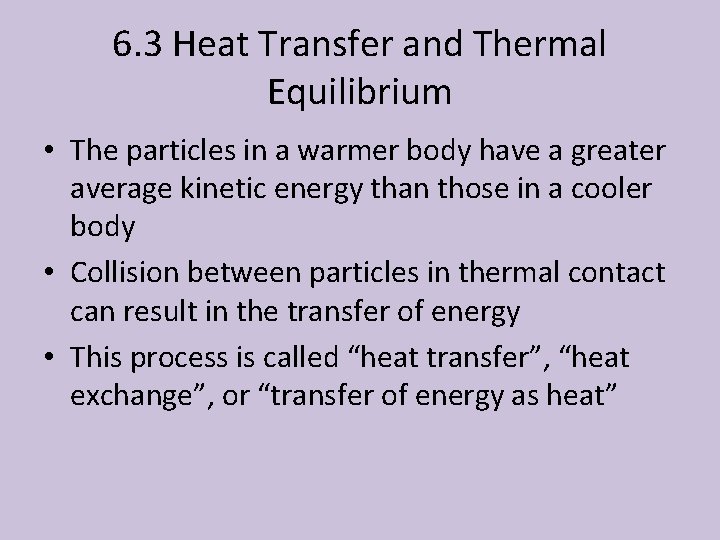
6. 3 Heat Transfer and Thermal Equilibrium • The particles in a warmer body have a greater average kinetic energy than those in a cooler body • Collision between particles in thermal contact can result in the transfer of energy • This process is called “heat transfer”, “heat exchange”, or “transfer of energy as heat”
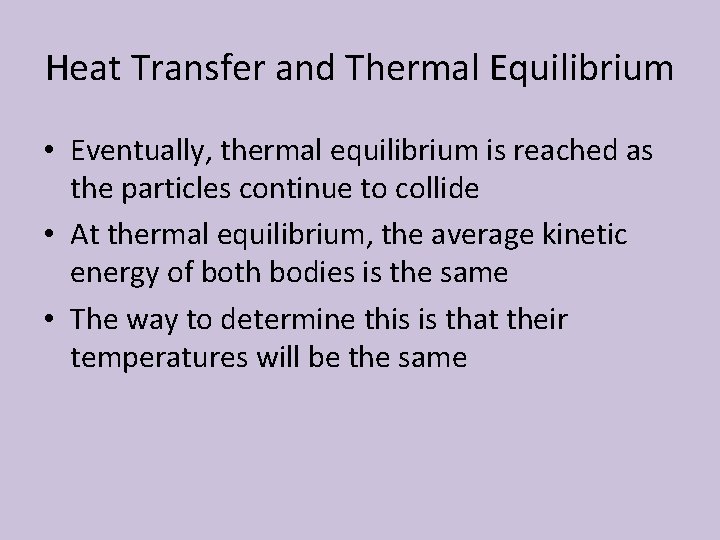
Heat Transfer and Thermal Equilibrium • Eventually, thermal equilibrium is reached as the particles continue to collide • At thermal equilibrium, the average kinetic energy of both bodies is the same • The way to determine this is that their temperatures will be the same
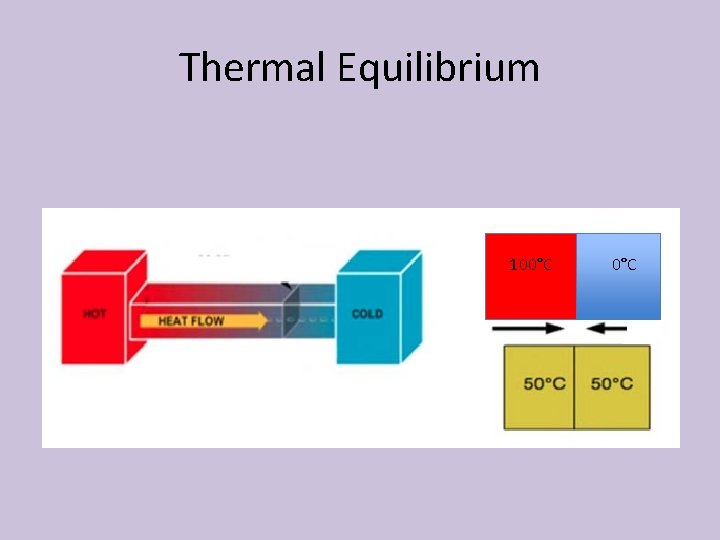
Thermal Equilibrium 100°C
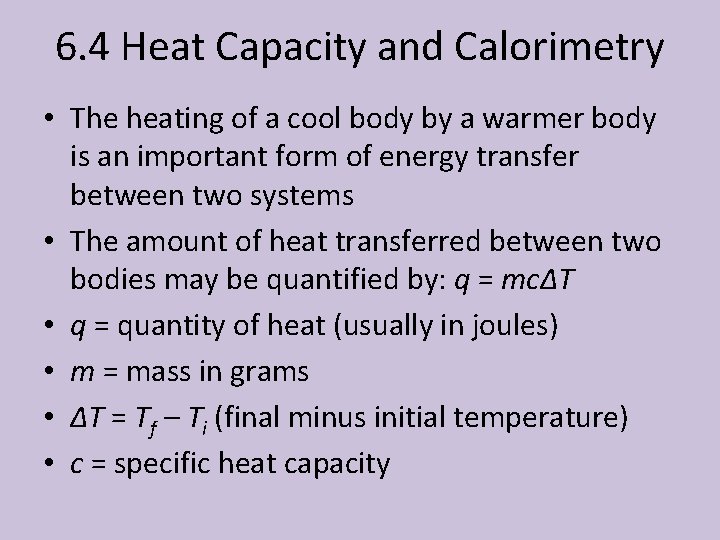
6. 4 Heat Capacity and Calorimetry • The heating of a cool body by a warmer body is an important form of energy transfer between two systems • The amount of heat transferred between two bodies may be quantified by: q = mcΔT • q = quantity of heat (usually in joules) • m = mass in grams • ΔT = Tf – Ti (final minus initial temperature) • c = specific heat capacity
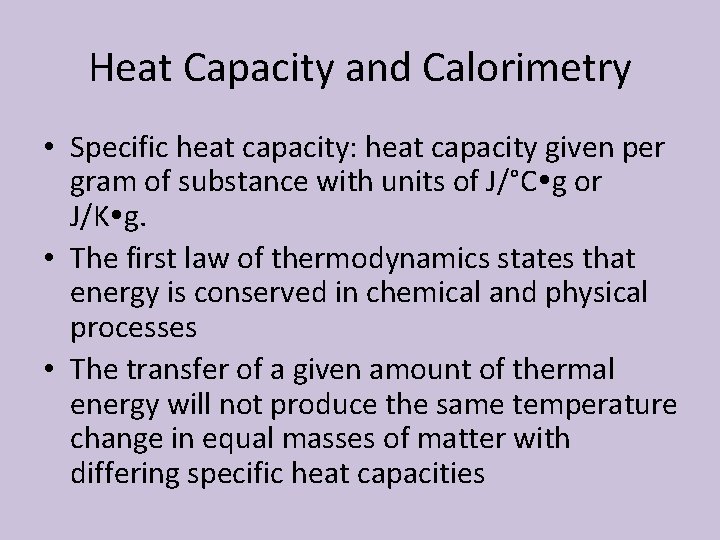
Heat Capacity and Calorimetry • Specific heat capacity: heat capacity given per gram of substance with units of J/°C g or J/K g. • The first law of thermodynamics states that energy is conserved in chemical and physical processes • The transfer of a given amount of thermal energy will not produce the same temperature change in equal masses of matter with differing specific heat capacities
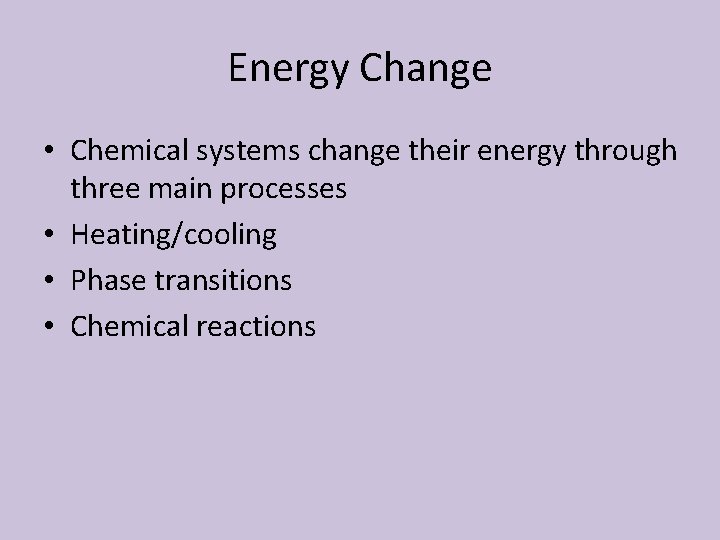
Energy Change • Chemical systems change their energy through three main processes • Heating/cooling • Phase transitions • Chemical reactions
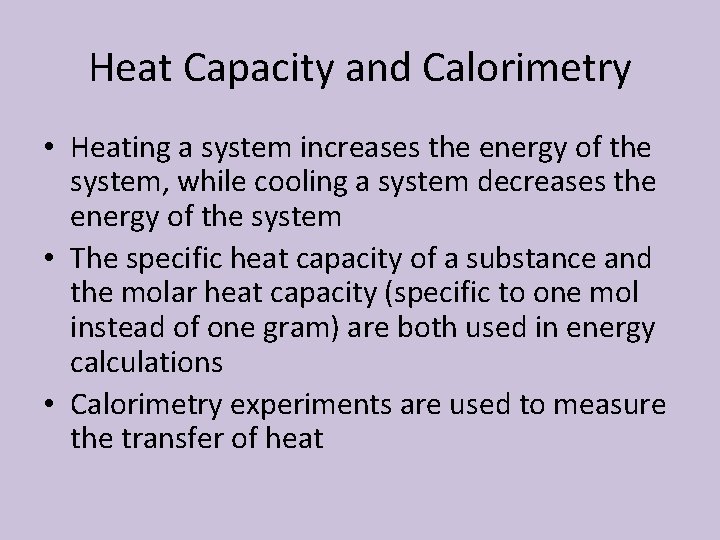
Heat Capacity and Calorimetry • Heating a system increases the energy of the system, while cooling a system decreases the energy of the system • The specific heat capacity of a substance and the molar heat capacity (specific to one mol instead of one gram) are both used in energy calculations • Calorimetry experiments are used to measure the transfer of heat
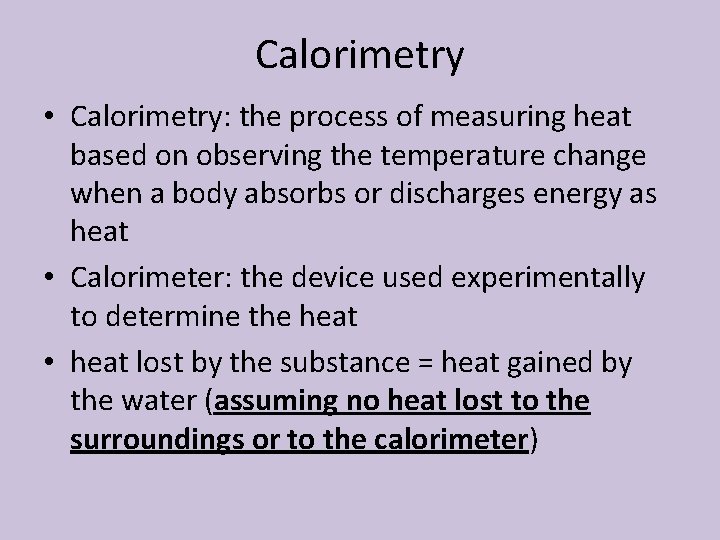
Calorimetry • Calorimetry: the process of measuring heat based on observing the temperature change when a body absorbs or discharges energy as heat • Calorimeter: the device used experimentally to determine the heat • heat lost by the substance = heat gained by the water (assuming no heat lost to the surroundings or to the calorimeter)
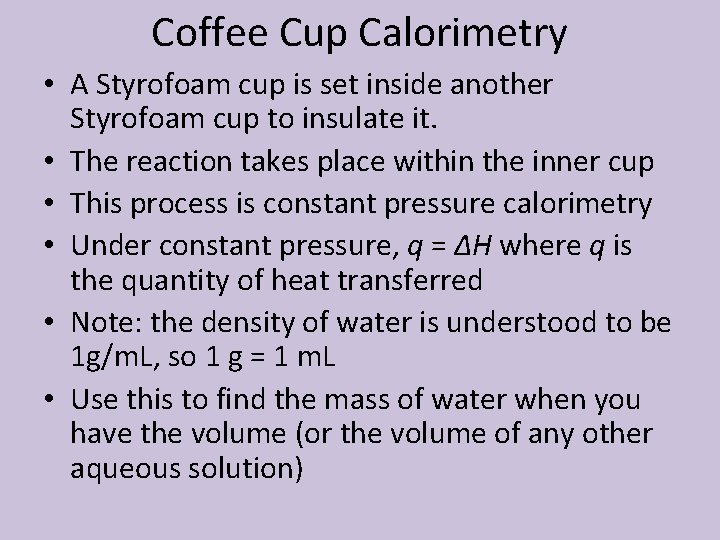
Coffee Cup Calorimetry • A Styrofoam cup is set inside another Styrofoam cup to insulate it. • The reaction takes place within the inner cup • This process is constant pressure calorimetry • Under constant pressure, q = ΔH where q is the quantity of heat transferred • Note: the density of water is understood to be 1 g/m. L, so 1 g = 1 m. L • Use this to find the mass of water when you have the volume (or the volume of any other aqueous solution)
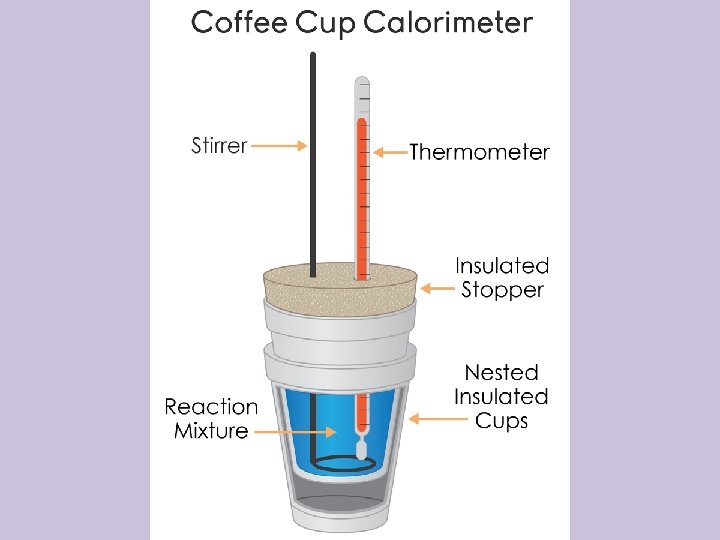
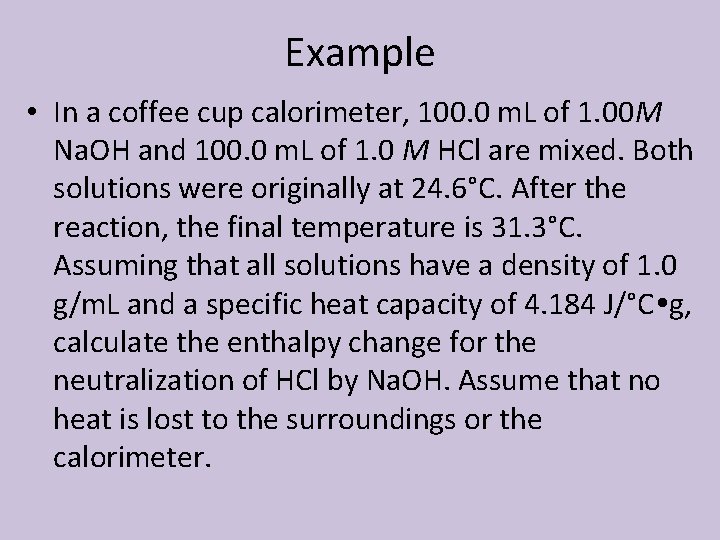
Example • In a coffee cup calorimeter, 100. 0 m. L of 1. 00 M Na. OH and 100. 0 m. L of 1. 0 M HCl are mixed. Both solutions were originally at 24. 6°C. After the reaction, the final temperature is 31. 3°C. Assuming that all solutions have a density of 1. 0 g/m. L and a specific heat capacity of 4. 184 J/°C g, calculate the enthalpy change for the neutralization of HCl by Na. OH. Assume that no heat is lost to the surroundings or the calorimeter.
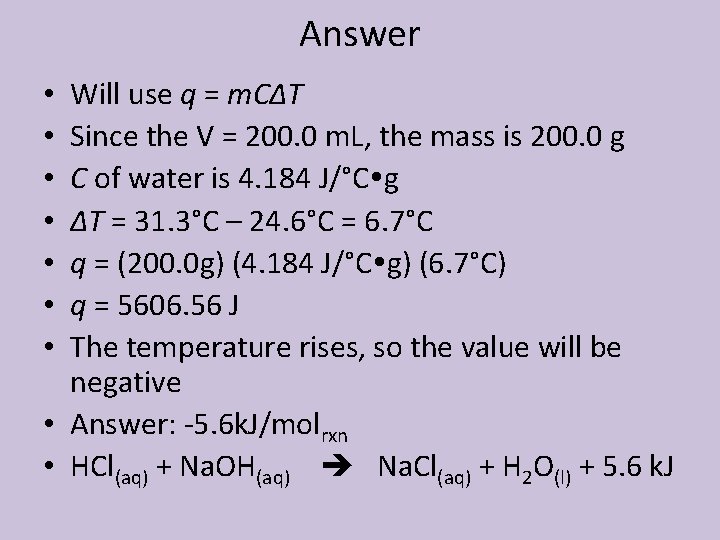
Answer Will use q = m. CΔT Since the V = 200. 0 m. L, the mass is 200. 0 g C of water is 4. 184 J/°C g ΔT = 31. 3°C – 24. 6°C = 6. 7°C q = (200. 0 g) (4. 184 J/°C g) (6. 7°C) q = 5606. 56 J The temperature rises, so the value will be negative • Answer: -5. 6 k. J/molrxn • HCl(aq) + Na. OH(aq) Na. Cl(aq) + H 2 O(l) + 5. 6 k. J • •
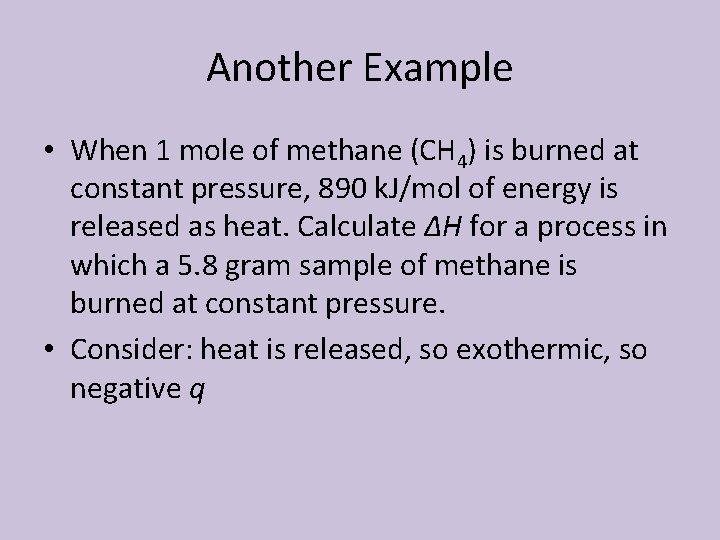
Another Example • When 1 mole of methane (CH 4) is burned at constant pressure, 890 k. J/mol of energy is released as heat. Calculate ΔH for a process in which a 5. 8 gram sample of methane is burned at constant pressure. • Consider: heat is released, so exothermic, so negative q
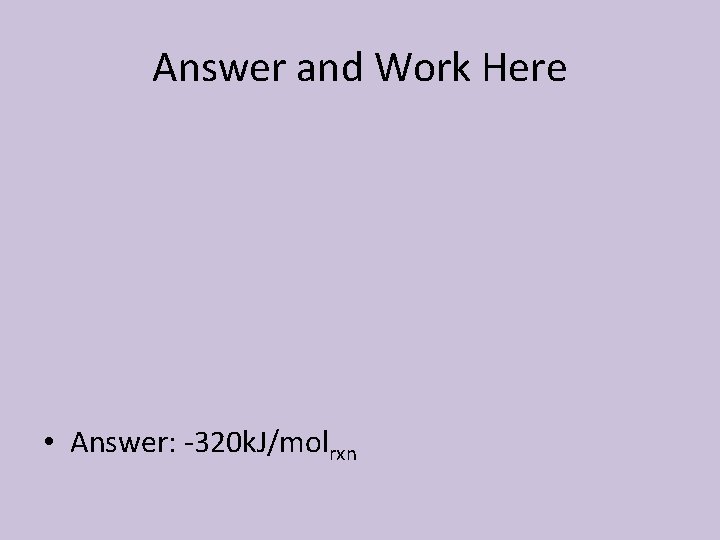
Answer and Work Here • Answer: -320 k. J/molrxn
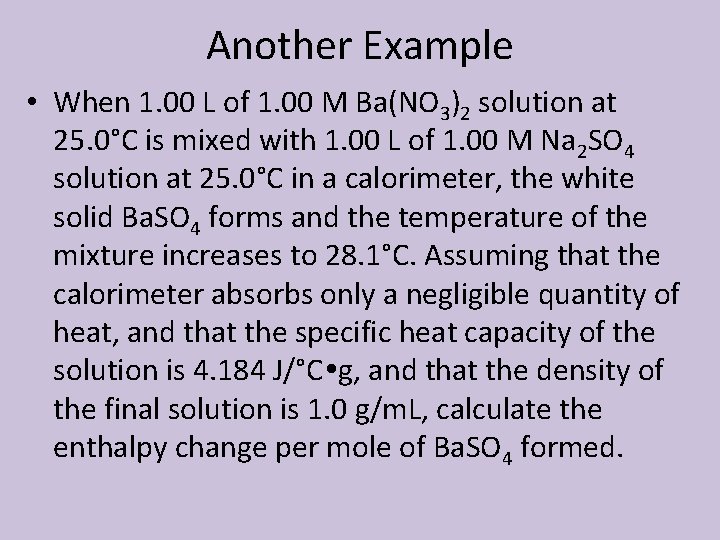
Another Example • When 1. 00 L of 1. 00 M Ba(NO 3)2 solution at 25. 0°C is mixed with 1. 00 L of 1. 00 M Na 2 SO 4 solution at 25. 0°C in a calorimeter, the white solid Ba. SO 4 forms and the temperature of the mixture increases to 28. 1°C. Assuming that the calorimeter absorbs only a negligible quantity of heat, and that the specific heat capacity of the solution is 4. 184 J/°C g, and that the density of the final solution is 1. 0 g/m. L, calculate the enthalpy change per mole of Ba. SO 4 formed.
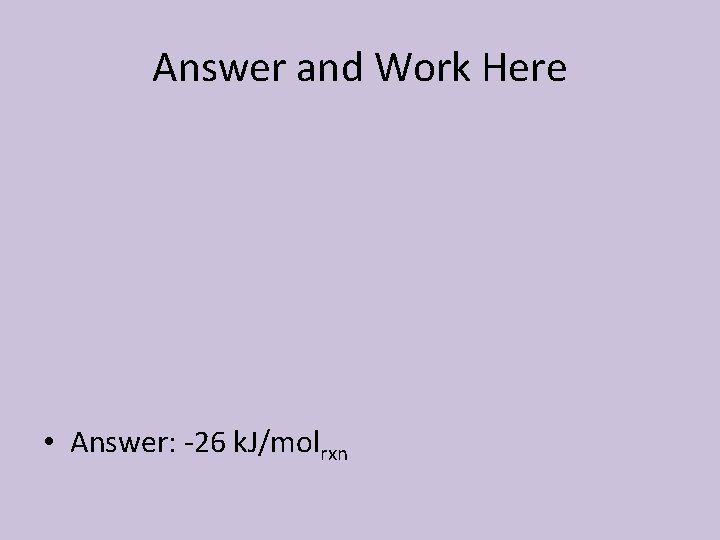
Answer and Work Here • Answer: -26 k. J/molrxn
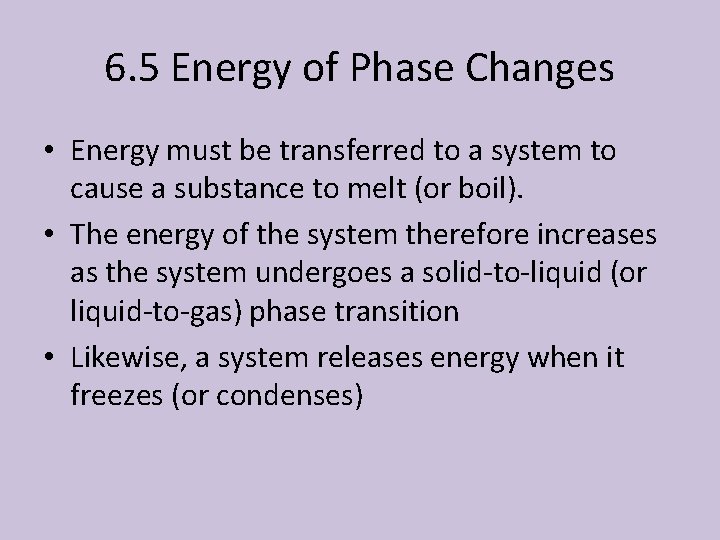
6. 5 Energy of Phase Changes • Energy must be transferred to a system to cause a substance to melt (or boil). • The energy of the system therefore increases as the system undergoes a solid-to-liquid (or liquid-to-gas) phase transition • Likewise, a system releases energy when it freezes (or condenses)
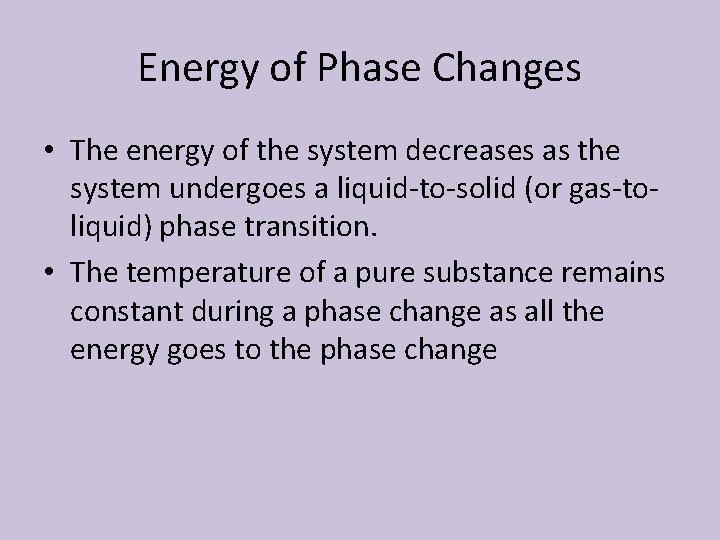
Energy of Phase Changes • The energy of the system decreases as the system undergoes a liquid-to-solid (or gas-toliquid) phase transition. • The temperature of a pure substance remains constant during a phase change as all the energy goes to the phase change
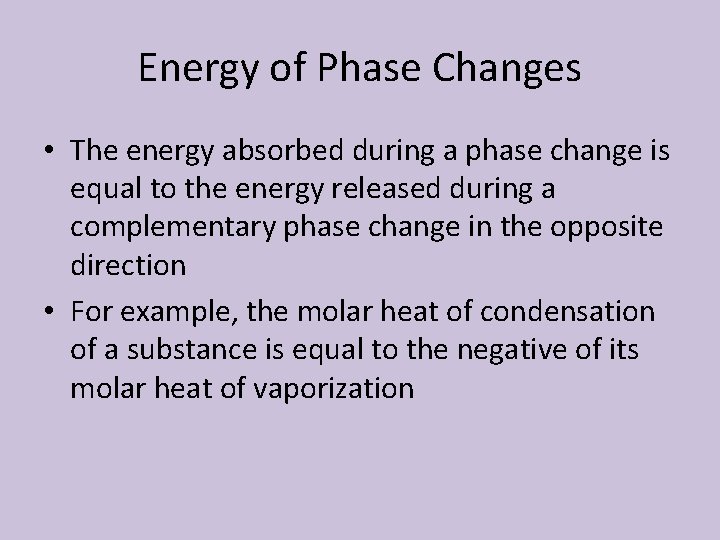
Energy of Phase Changes • The energy absorbed during a phase change is equal to the energy released during a complementary phase change in the opposite direction • For example, the molar heat of condensation of a substance is equal to the negative of its molar heat of vaporization
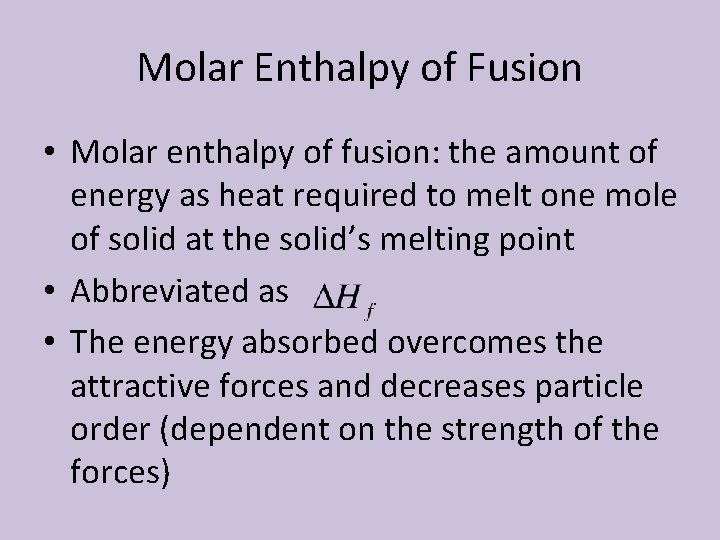
Molar Enthalpy of Fusion • Molar enthalpy of fusion: the amount of energy as heat required to melt one mole of solid at the solid’s melting point • Abbreviated as • The energy absorbed overcomes the attractive forces and decreases particle order (dependent on the strength of the forces)
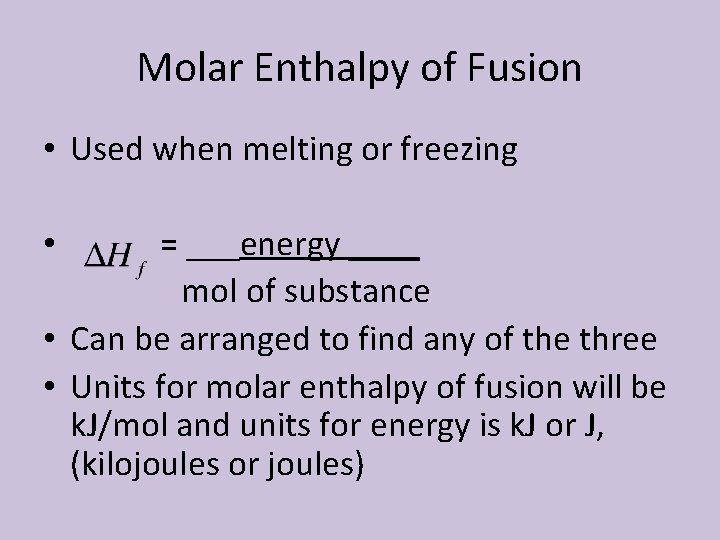
Molar Enthalpy of Fusion • Used when melting or freezing = ___energy ____ mol of substance • Can be arranged to find any of the three • Units for molar enthalpy of fusion will be k. J/mol and units for energy is k. J or J, (kilojoules or joules) •
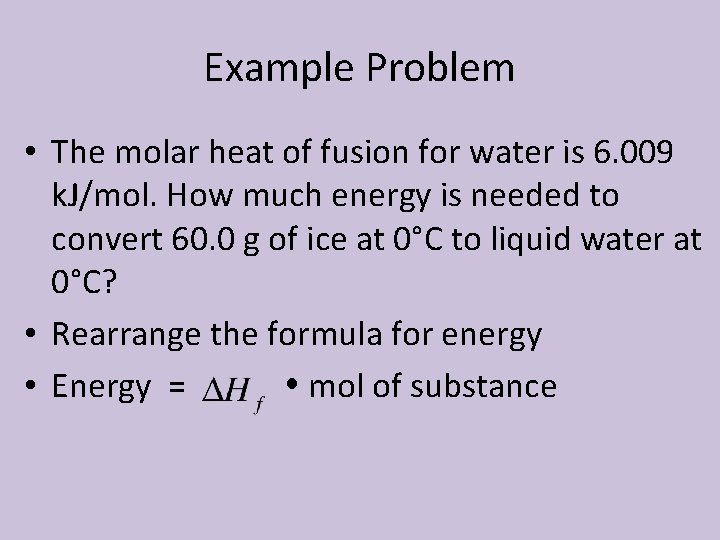
Example Problem • The molar heat of fusion for water is 6. 009 k. J/mol. How much energy is needed to convert 60. 0 g of ice at 0°C to liquid water at 0°C? • Rearrange the formula for energy • Energy = mol of substance
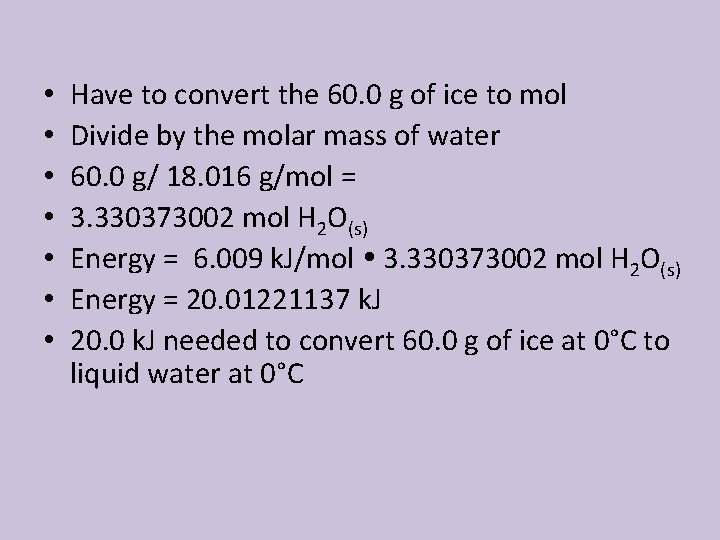
• • Have to convert the 60. 0 g of ice to mol Divide by the molar mass of water 60. 0 g/ 18. 016 g/mol = 3. 330373002 mol H 2 O(s) Energy = 6. 009 k. J/mol 3. 330373002 mol H 2 O(s) Energy = 20. 01221137 k. J 20. 0 k. J needed to convert 60. 0 g of ice at 0°C to liquid water at 0°C
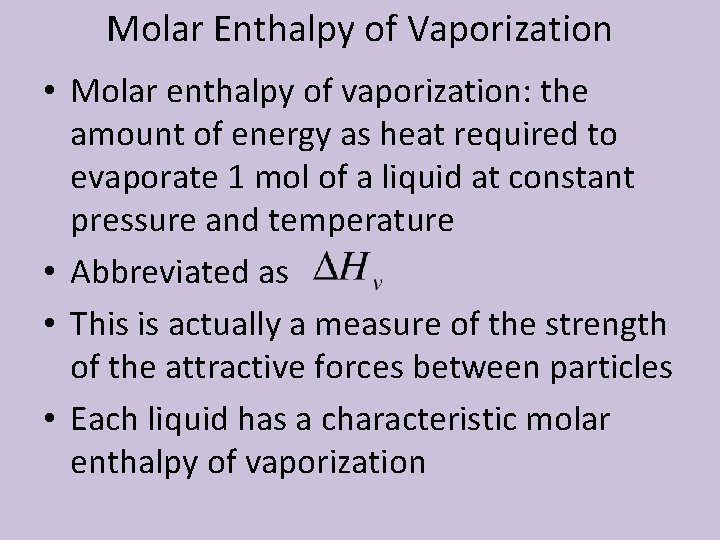
Molar Enthalpy of Vaporization • Molar enthalpy of vaporization: the amount of energy as heat required to evaporate 1 mol of a liquid at constant pressure and temperature • Abbreviated as • This is actually a measure of the strength of the attractive forces between particles • Each liquid has a characteristic molar enthalpy of vaporization
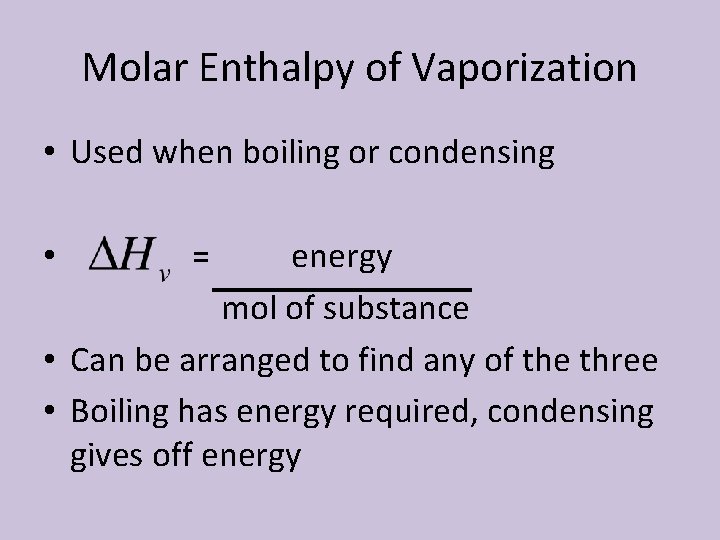
Molar Enthalpy of Vaporization • Used when boiling or condensing energy mol of substance • Can be arranged to find any of the three • Boiling has energy required, condensing gives off energy • =
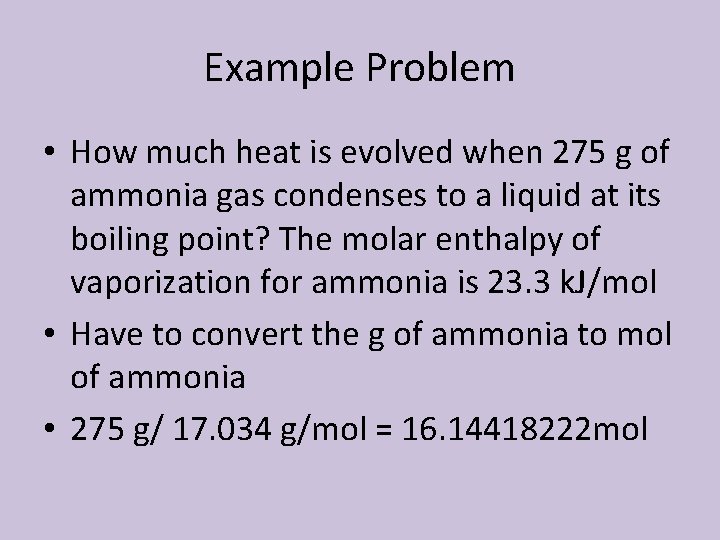
Example Problem • How much heat is evolved when 275 g of ammonia gas condenses to a liquid at its boiling point? The molar enthalpy of vaporization for ammonia is 23. 3 k. J/mol • Have to convert the g of ammonia to mol of ammonia • 275 g/ 17. 034 g/mol = 16. 14418222 mol
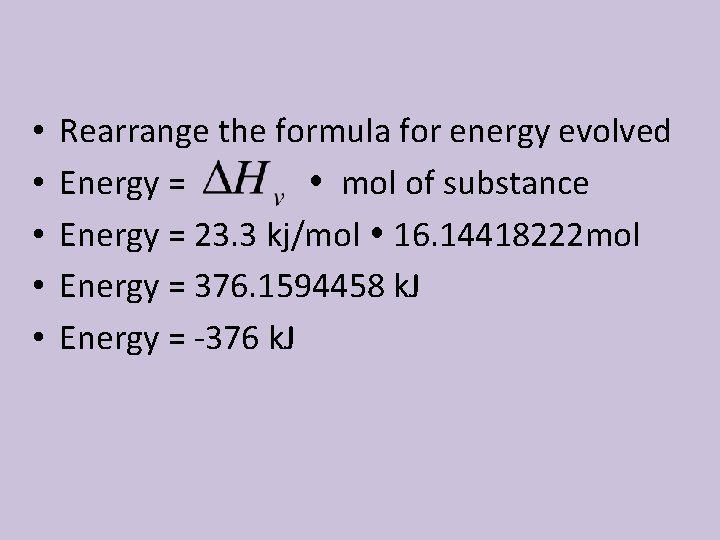
• • • Rearrange the formula for energy evolved Energy = mol of substance Energy = 23. 3 kj/mol 16. 14418222 mol Energy = 376. 1594458 k. J Energy = -376 k. J
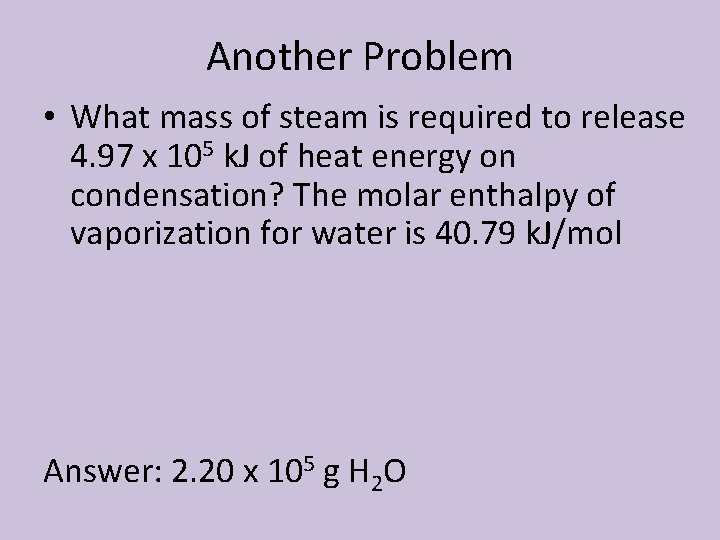
Another Problem • What mass of steam is required to release 4. 97 x 105 k. J of heat energy on condensation? The molar enthalpy of vaporization for water is 40. 79 k. J/mol Answer: 2. 20 x 105 g H 2 O
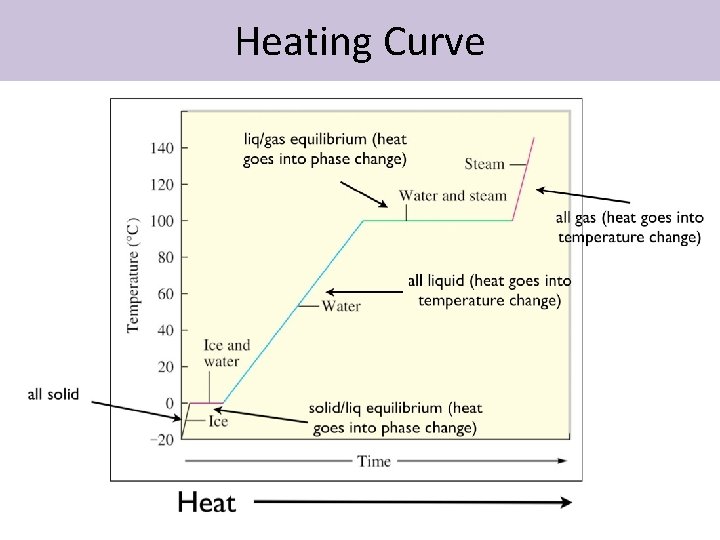
Heating Curve
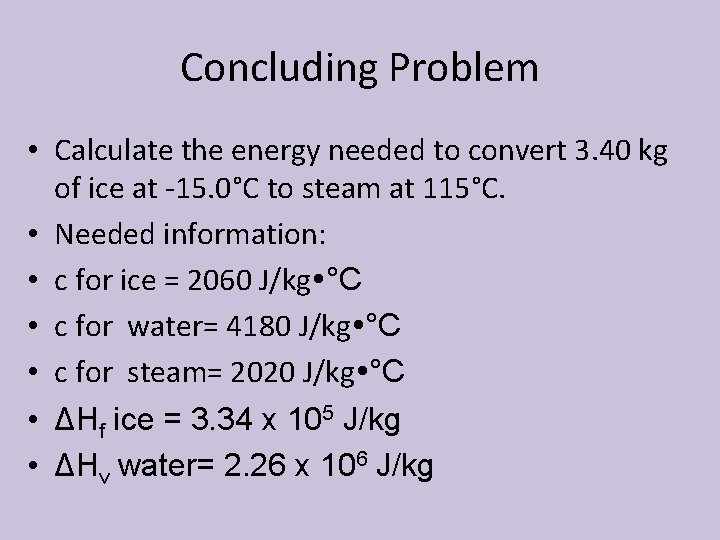
Concluding Problem • Calculate the energy needed to convert 3. 40 kg of ice at -15. 0°C to steam at 115°C. • Needed information: • c for ice = 2060 J/kg °C • c for water= 4180 J/kg °C • c for steam= 2020 J/kg °C • ΔHf ice = 3. 34 x 105 J/kg • ΔHv water= 2. 26 x 106 J/kg
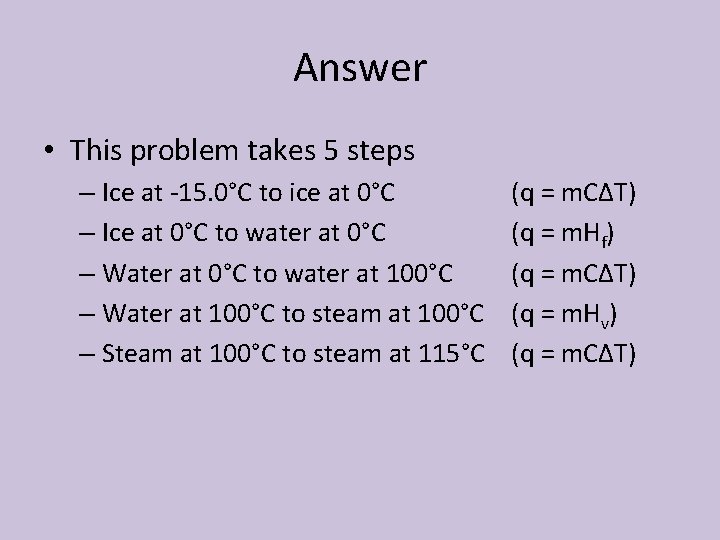
Answer • This problem takes 5 steps – Ice at -15. 0°C to ice at 0°C – Ice at 0°C to water at 0°C – Water at 0°C to water at 100°C – Water at 100°C to steam at 100°C – Steam at 100°C to steam at 115°C (q = m. CΔT) (q = m. Hf) (q = m. CΔT) (q = m. Hv) (q = m. CΔT)
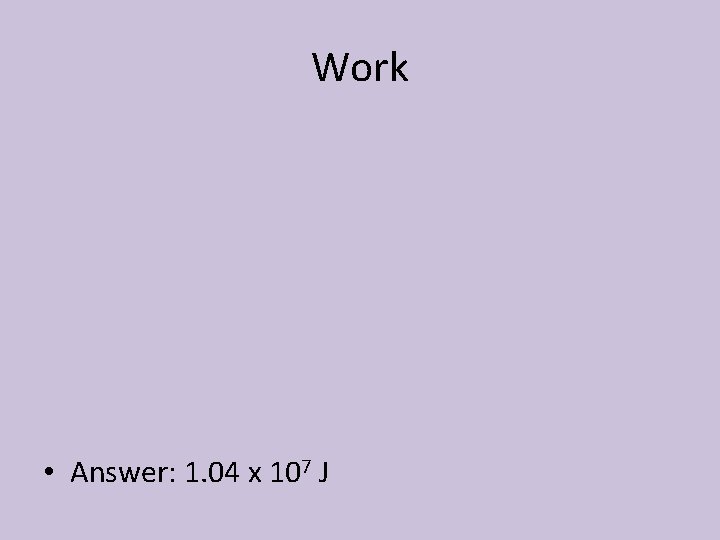
Work • Answer: 1. 04 x 107 J
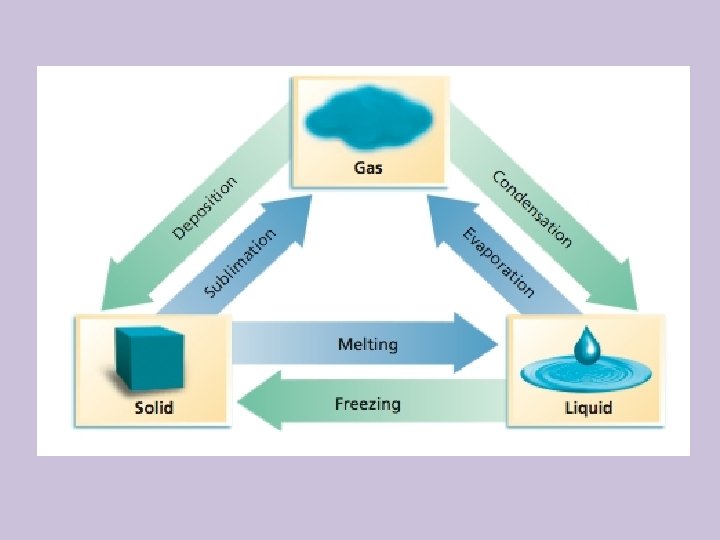
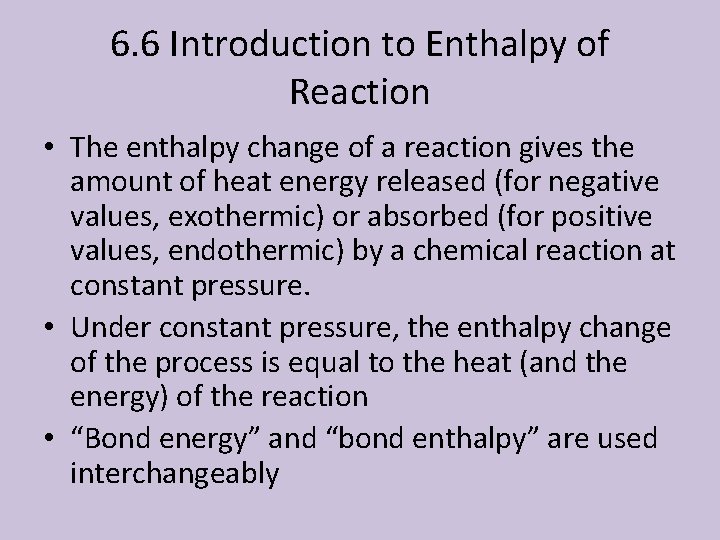
6. 6 Introduction to Enthalpy of Reaction • The enthalpy change of a reaction gives the amount of heat energy released (for negative values, exothermic) or absorbed (for positive values, endothermic) by a chemical reaction at constant pressure. • Under constant pressure, the enthalpy change of the process is equal to the heat (and the energy) of the reaction • “Bond energy” and “bond enthalpy” are used interchangeably
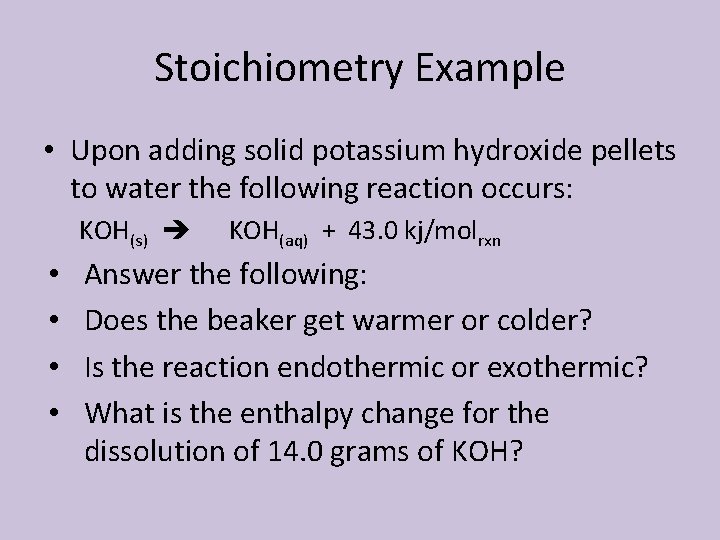
Stoichiometry Example • Upon adding solid potassium hydroxide pellets to water the following reaction occurs: KOH(s) • • KOH(aq) + 43. 0 kj/molrxn Answer the following: Does the beaker get warmer or colder? Is the reaction endothermic or exothermic? What is the enthalpy change for the dissolution of 14. 0 grams of KOH?
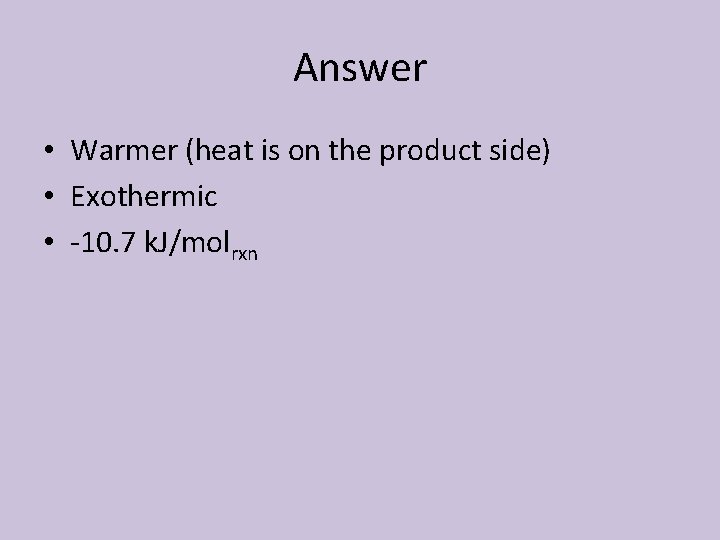
Answer • Warmer (heat is on the product side) • Exothermic • -10. 7 k. J/molrxn
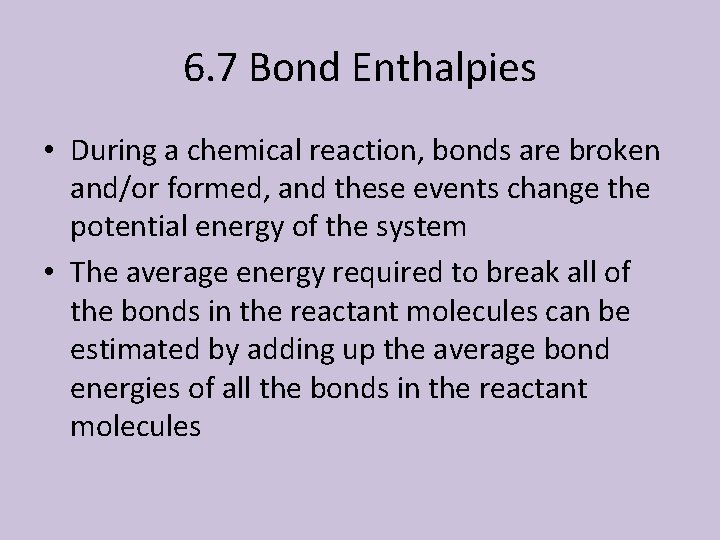
6. 7 Bond Enthalpies • During a chemical reaction, bonds are broken and/or formed, and these events change the potential energy of the system • The average energy required to break all of the bonds in the reactant molecules can be estimated by adding up the average bond energies of all the bonds in the reactant molecules
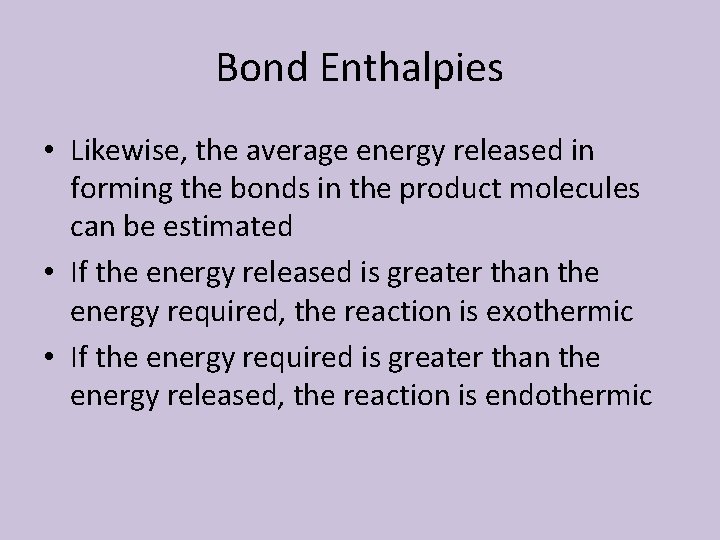
Bond Enthalpies • Likewise, the average energy released in forming the bonds in the product molecules can be estimated • If the energy released is greater than the energy required, the reaction is exothermic • If the energy required is greater than the energy released, the reaction is endothermic
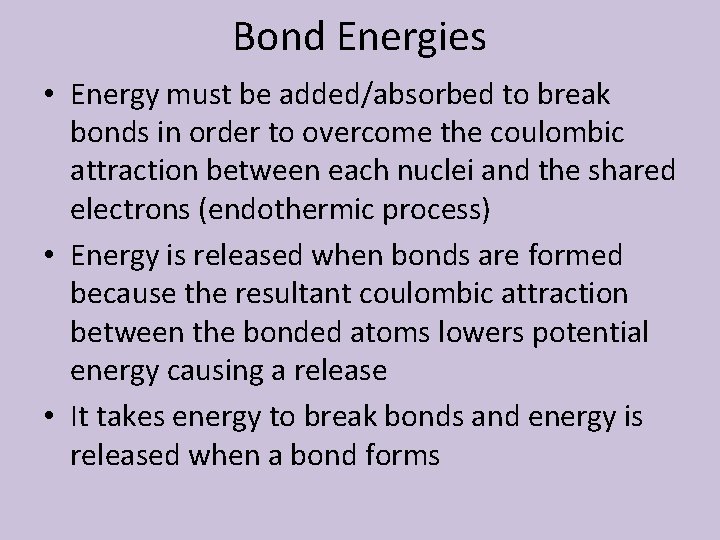
Bond Energies • Energy must be added/absorbed to break bonds in order to overcome the coulombic attraction between each nuclei and the shared electrons (endothermic process) • Energy is released when bonds are formed because the resultant coulombic attraction between the bonded atoms lowers potential energy causing a release • It takes energy to break bonds and energy is released when a bond forms
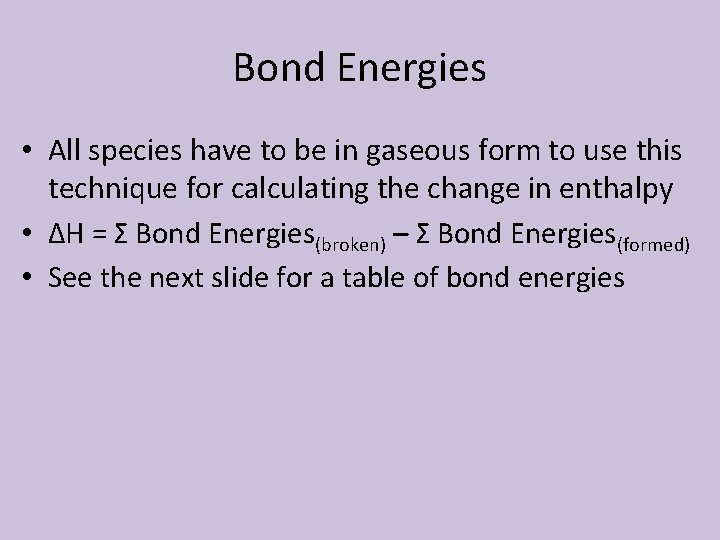
Bond Energies • All species have to be in gaseous form to use this technique for calculating the change in enthalpy • ΔH = Σ Bond Energies(broken) – Σ Bond Energies(formed) • See the next slide for a table of bond energies
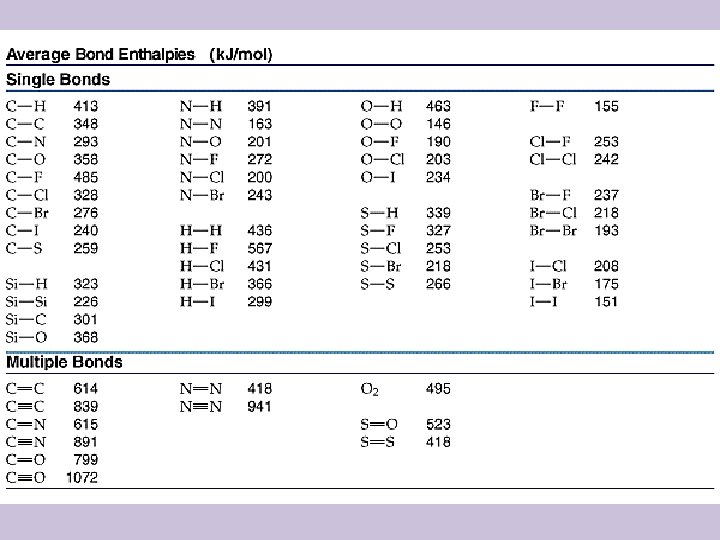
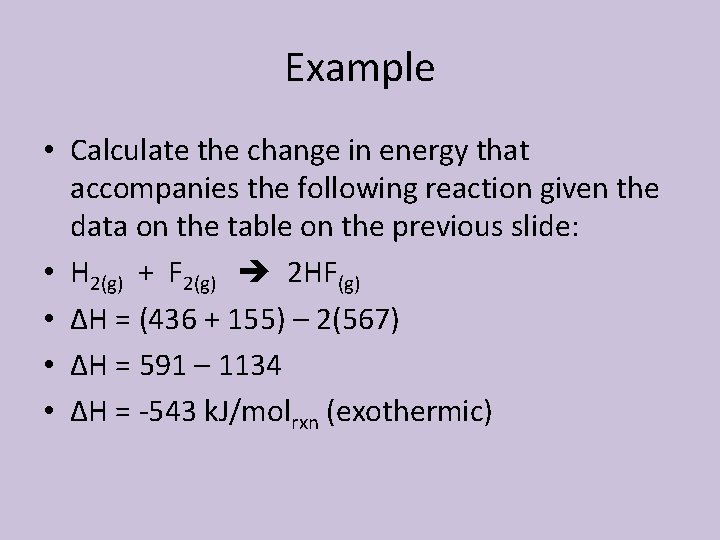
Example • Calculate the change in energy that accompanies the following reaction given the data on the table on the previous slide: • H 2(g) + F 2(g) 2 HF(g) • ΔH = (436 + 155) – 2(567) • ΔH = 591 – 1134 • ΔH = -543 k. J/molrxn (exothermic)
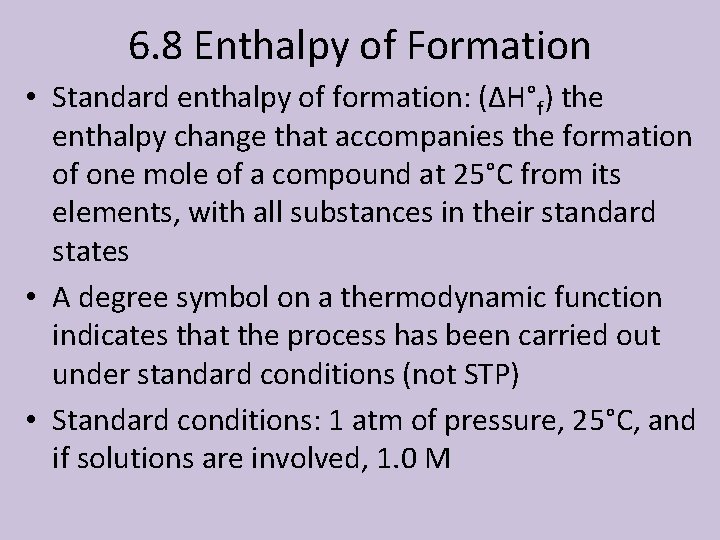
6. 8 Enthalpy of Formation • Standard enthalpy of formation: (ΔH°f) the enthalpy change that accompanies the formation of one mole of a compound at 25°C from its elements, with all substances in their standard states • A degree symbol on a thermodynamic function indicates that the process has been carried out under standard conditions (not STP) • Standard conditions: 1 atm of pressure, 25°C, and if solutions are involved, 1. 0 M
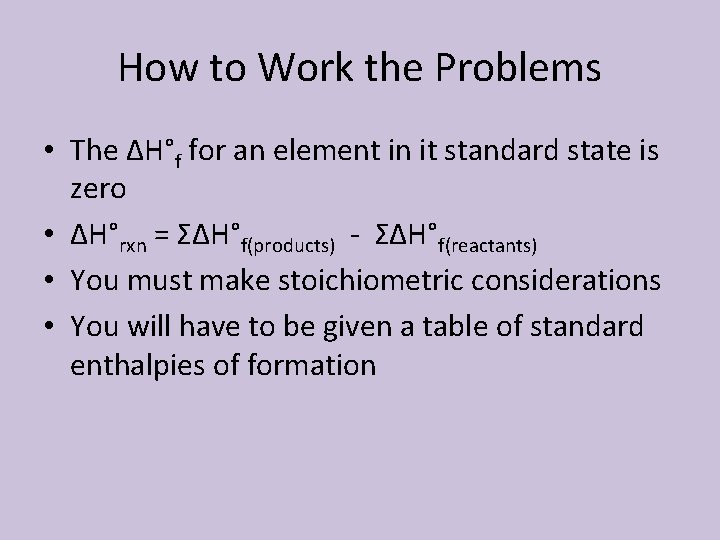
How to Work the Problems • The ΔH°f for an element in it standard state is zero • ΔH°rxn = ΣΔH°f(products) - ΣΔH°f(reactants) • You must make stoichiometric considerations • You will have to be given a table of standard enthalpies of formation
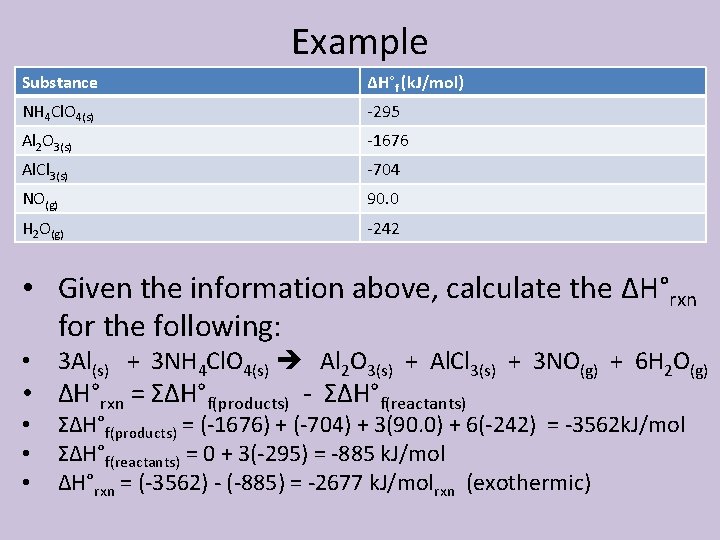
Example Substance ΔH°f (k. J/mol) NH 4 Cl. O 4(s) -295 Al 2 O 3(s) -1676 Al. Cl 3(s) -704 NO(g) 90. 0 H 2 O(g) -242 • Given the information above, calculate the ΔH°rxn for the following: • 3 Al(s) + 3 NH 4 Cl. O 4(s) Al 2 O 3(s) + Al. Cl 3(s) + 3 NO(g) + 6 H 2 O(g) • ΔH°rxn = ΣΔH°f(products) - ΣΔH°f(reactants) • • • ΣΔH°f(products) = (-1676) + (-704) + 3(90. 0) + 6(-242) = -3562 k. J/mol ΣΔH°f(reactants) = 0 + 3(-295) = -885 k. J/mol ΔH°rxn = (-3562) - (-885) = -2677 k. J/molrxn (exothermic)
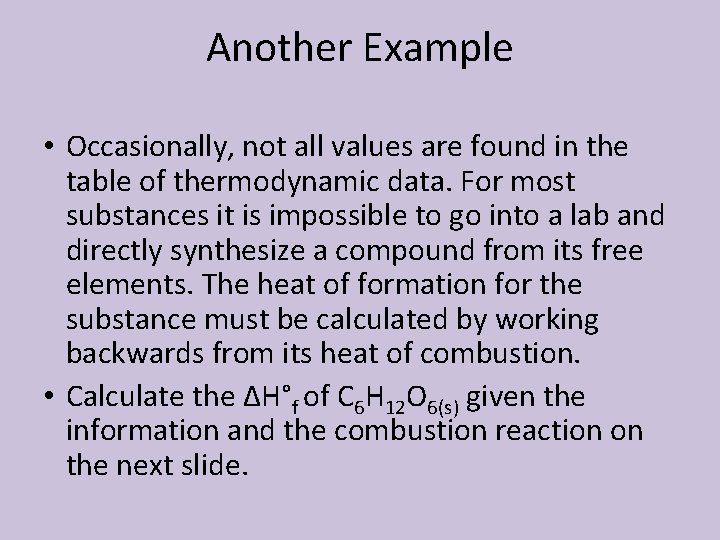
Another Example • Occasionally, not all values are found in the table of thermodynamic data. For most substances it is impossible to go into a lab and directly synthesize a compound from its free elements. The heat of formation for the substance must be calculated by working backwards from its heat of combustion. • Calculate the ΔH°f of C 6 H 12 O 6(s) given the information and the combustion reaction on the next slide.
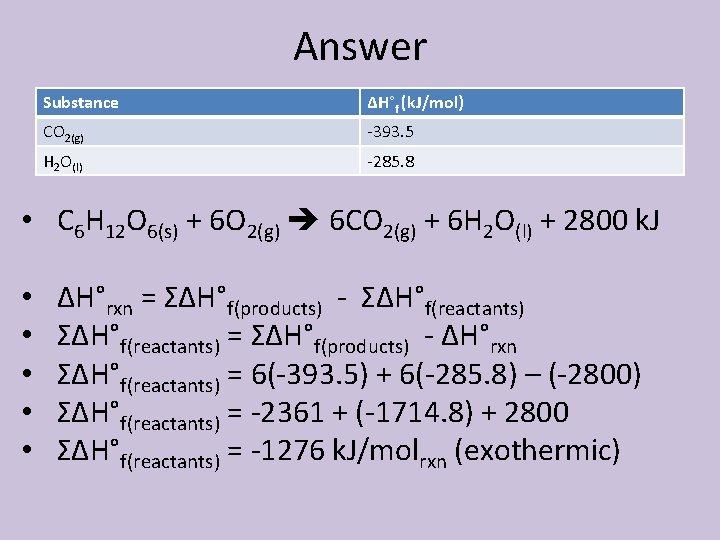
Answer Substance ΔH°f (k. J/mol) CO 2(g) -393. 5 H 2 O(l) -285. 8 • C 6 H 12 O 6(s) + 6 O 2(g) 6 CO 2(g) + 6 H 2 O(l) + 2800 k. J • • • ΔH°rxn = ΣΔH°f(products) - ΣΔH°f(reactants) = ΣΔH°f(products) - ΔH°rxn ΣΔH°f(reactants) = 6(-393. 5) + 6(-285. 8) – (-2800) ΣΔH°f(reactants) = -2361 + (-1714. 8) + 2800 ΣΔH°f(reactants) = -1276 k. J/molrxn (exothermic)
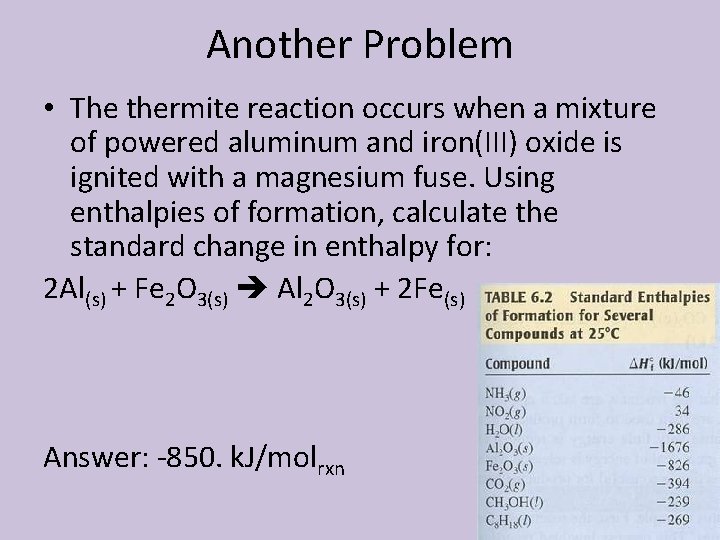
Another Problem • The thermite reaction occurs when a mixture of powered aluminum and iron(III) oxide is ignited with a magnesium fuse. Using enthalpies of formation, calculate the standard change in enthalpy for: 2 Al(s) + Fe 2 O 3(s) Al 2 O 3(s) + 2 Fe(s) Answer: -850. k. J/molrxn
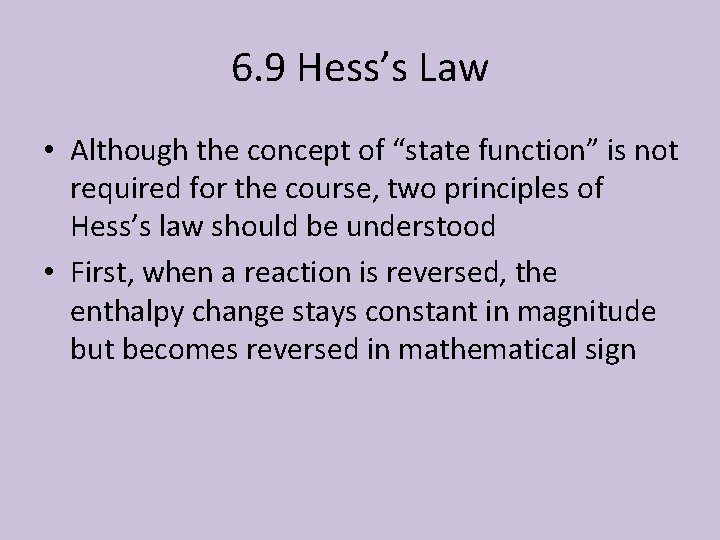
6. 9 Hess’s Law • Although the concept of “state function” is not required for the course, two principles of Hess’s law should be understood • First, when a reaction is reversed, the enthalpy change stays constant in magnitude but becomes reversed in mathematical sign
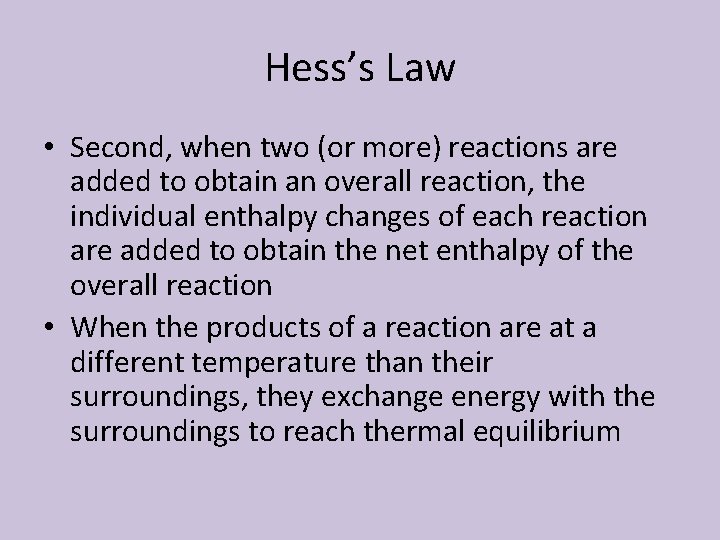
Hess’s Law • Second, when two (or more) reactions are added to obtain an overall reaction, the individual enthalpy changes of each reaction are added to obtain the net enthalpy of the overall reaction • When the products of a reaction are at a different temperature than their surroundings, they exchange energy with the surroundings to reach thermal equilibrium
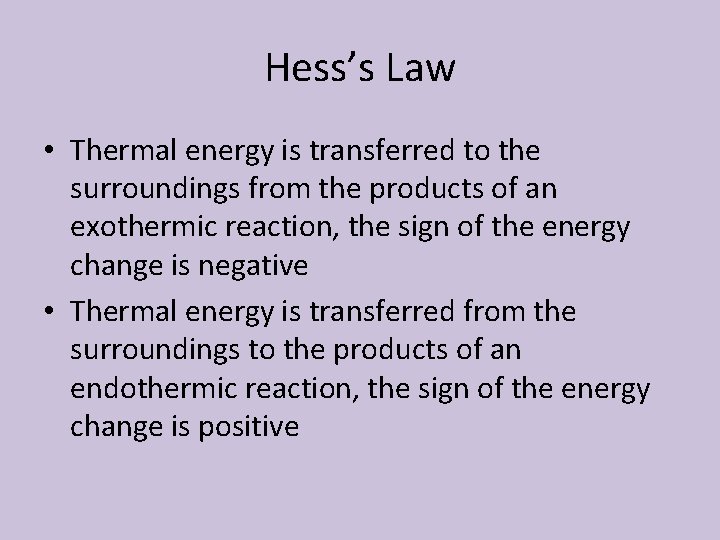
Hess’s Law • Thermal energy is transferred to the surroundings from the products of an exothermic reaction, the sign of the energy change is negative • Thermal energy is transferred from the surroundings to the products of an endothermic reaction, the sign of the energy change is positive
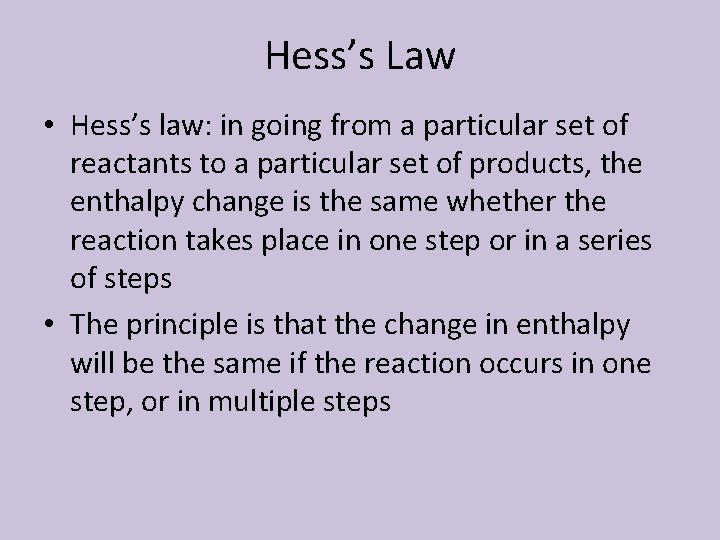
Hess’s Law • Hess’s law: in going from a particular set of reactants to a particular set of products, the enthalpy change is the same whether the reaction takes place in one step or in a series of steps • The principle is that the change in enthalpy will be the same if the reaction occurs in one step, or in multiple steps
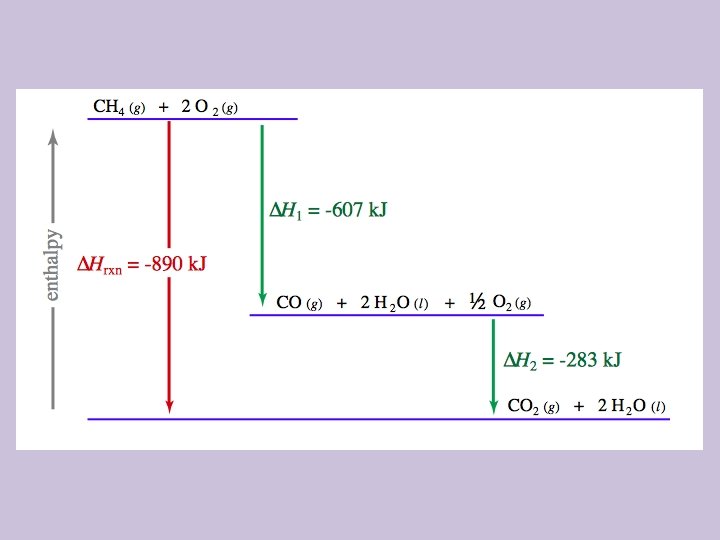
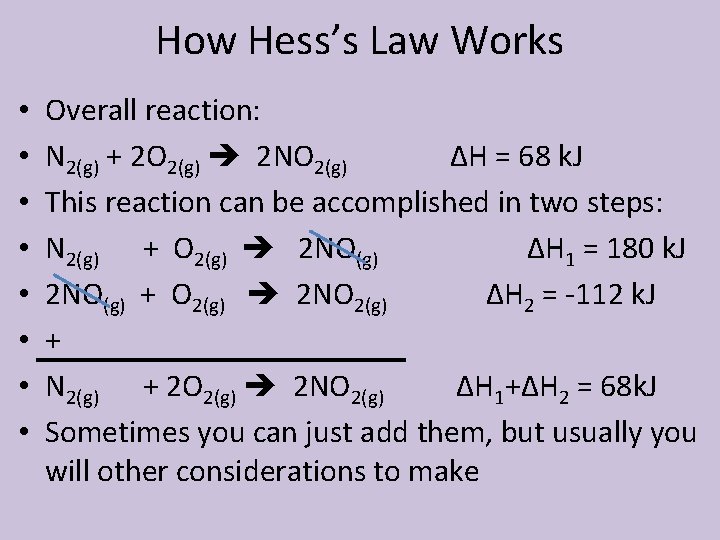
How Hess’s Law Works • • Overall reaction: N 2(g) + 2 O 2(g) 2 NO 2(g) ΔH = 68 k. J This reaction can be accomplished in two steps: N 2(g) + O 2(g) 2 NO(g) ΔH 1 = 180 k. J 2 NO(g) + O 2(g) 2 NO 2(g) ΔH 2 = -112 k. J + N 2(g) + 2 O 2(g) 2 NO 2(g) ΔH 1+ΔH 2 = 68 k. J Sometimes you can just add them, but usually you will other considerations to make
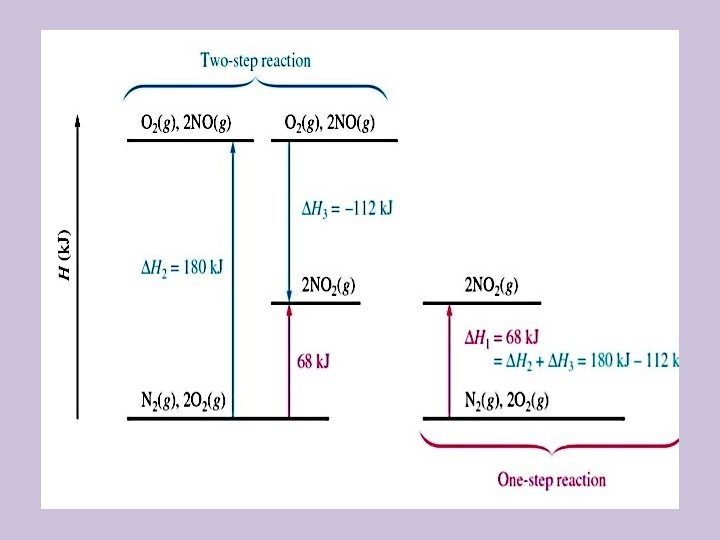
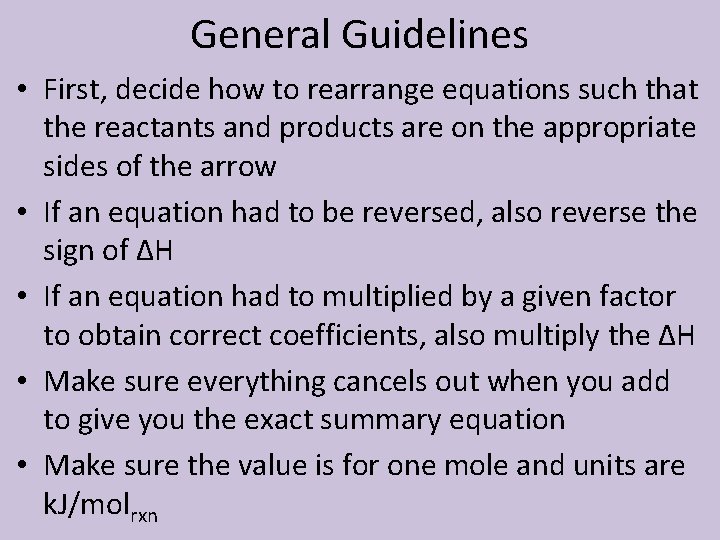
General Guidelines • First, decide how to rearrange equations such that the reactants and products are on the appropriate sides of the arrow • If an equation had to be reversed, also reverse the sign of ΔH • If an equation had to multiplied by a given factor to obtain correct coefficients, also multiply the ΔH • Make sure everything cancels out when you add to give you the exact summary equation • Make sure the value is for one mole and units are k. J/molrxn
Endothermic and exothermic reaction experiment grade 11
Endothermic and exothermic worksheet
Exothermic and endothermic homework
Endotermik tepkime
Is vaporization endothermic or exothermic
Endothermic vs exothermic
Methane oxygen endothermic or exothermic
Exothermic v endothermic
Is vaporization endothermic or exothermic
How to determine exothermic or endothermic
Examples of ectotherms
Cellular respiration endothermic or exothermic
Exothermic vs endothermic
Exothermic or endothermic
Exothermic vs endothermic
Melting freezing evaporation condensation sublimation
Endothermic energy
Is the combustion of propane endothermic or exothermic
Endo exo graph
Is a firecracker exploding endothermic or exothermic
Is photosynthesis exothermic
Your car burns gasoline as you drive from home to school
Phase change diagram endothermic exothermic
Endothermic enthalpy
Lesson 10 thermodynamics unit review
Endothermic and endergonic
Unit 6 review questions
Exothermic electron affinity trend
Whats the trend for atomic radius
Exothermic examples
Exothermic reaction increase temperature equilibrium
In exothermic reaction temperature
Exothermic reaction increase temperature equilibrium
Exothermic reaction increase temperature
Chemical reaction examples
Whats an exothermic reaction
Define ectothermic
Exothermic chemicals
Exothermic
Exothermic examples
Exothermic atmosphere furnace
Exothermic reaction
Nitrogen triiodide decomposition equation
Exothermic reaction
Label each step as endo or exothermic.
Exothermic
Changes of matter examples
Endothermic characteristics
Define endothermic
Labeled potential energy diagram
Endothermic animals with hair
Endothermic reaction for kids
Low ph alkaline waves have a ph of
Endothermic insects
Q in thermodynamics
Endothermic
Is endothermic positive or negative
Endothermic enthalpy
Enthalpy exo or endo
Are agnatha endothermic or ectothermic
Curtin-hammett principle
çengel
Introduction and basic concepts of thermodynamics
Reversible and irreversible processes in thermodynamics
Thermodynamics introduction and basic concepts