MondayTuesday Thermodynamics of aqueous solutions Ion association Pitzer
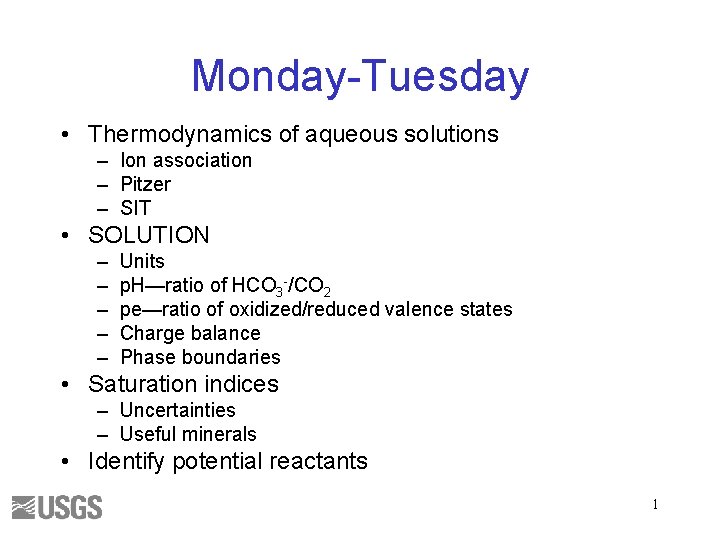
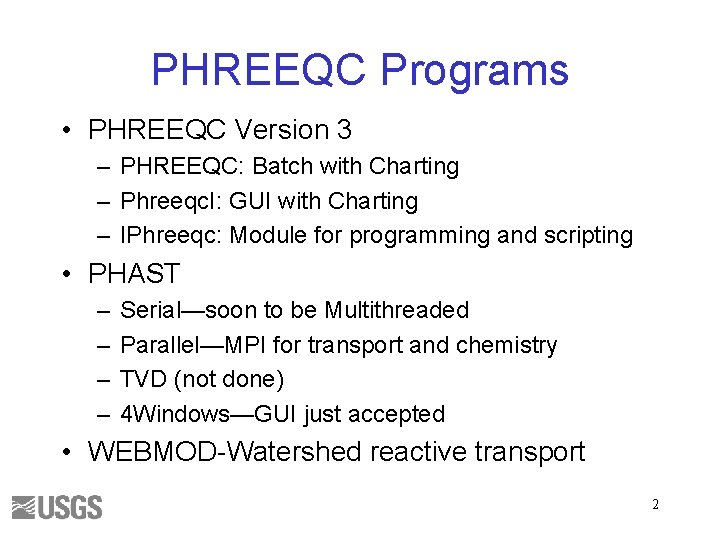
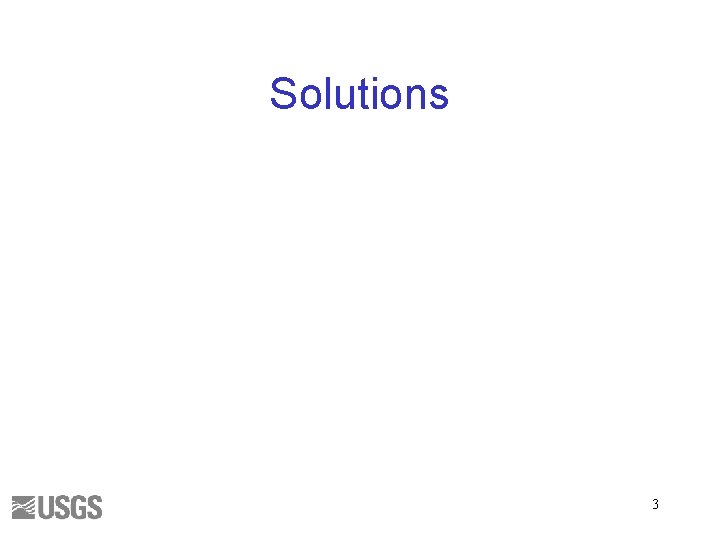
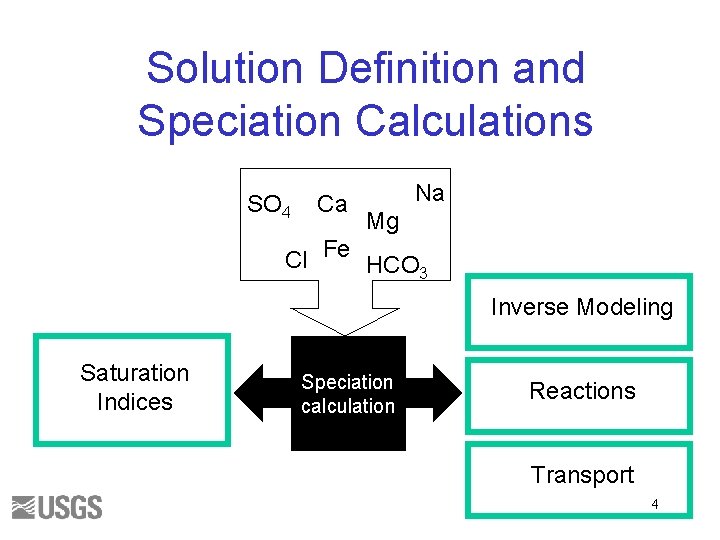
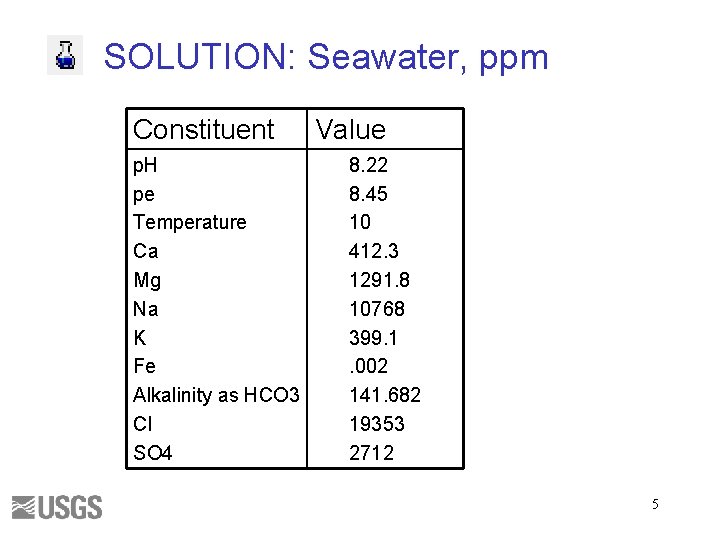
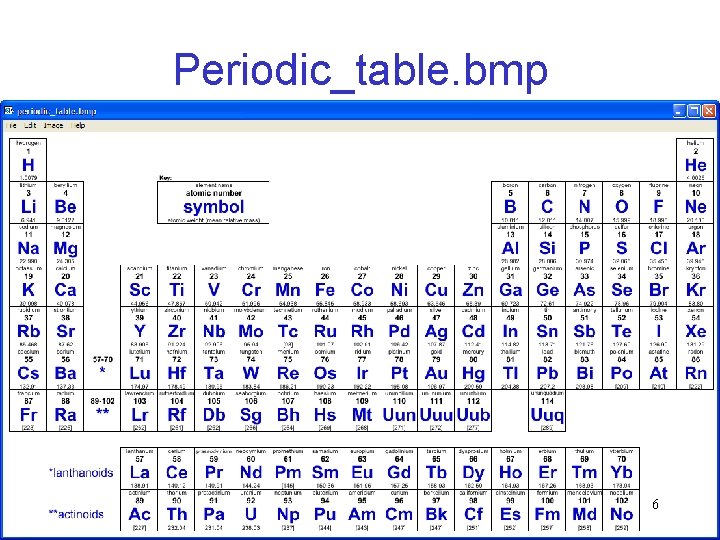
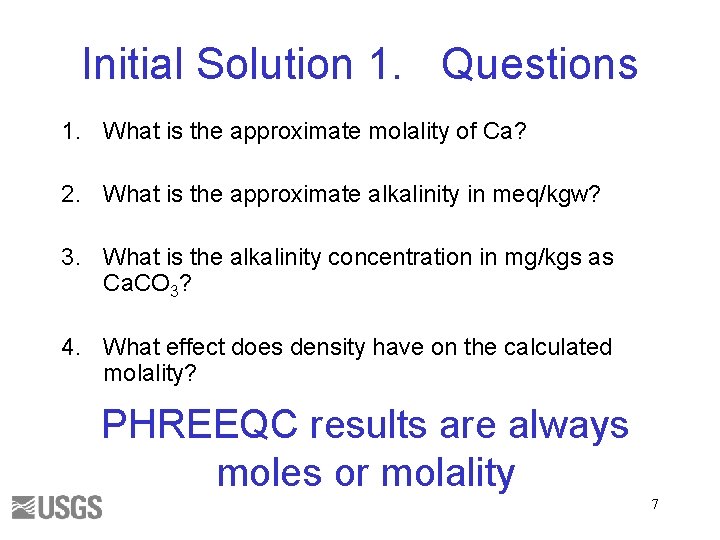
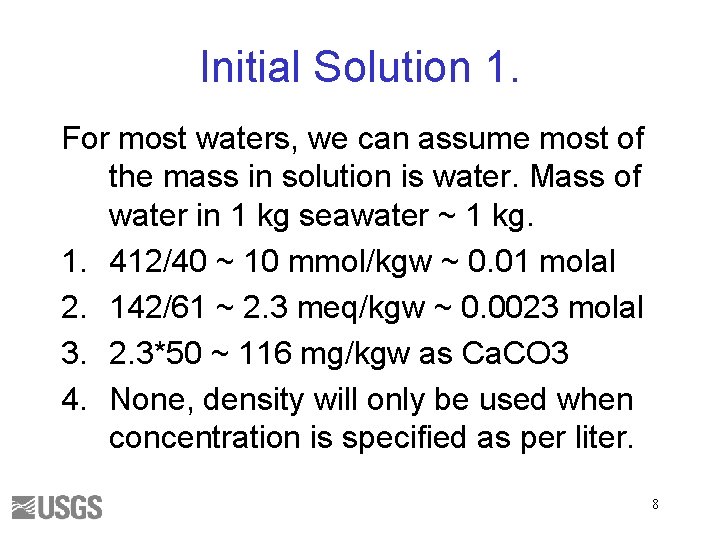
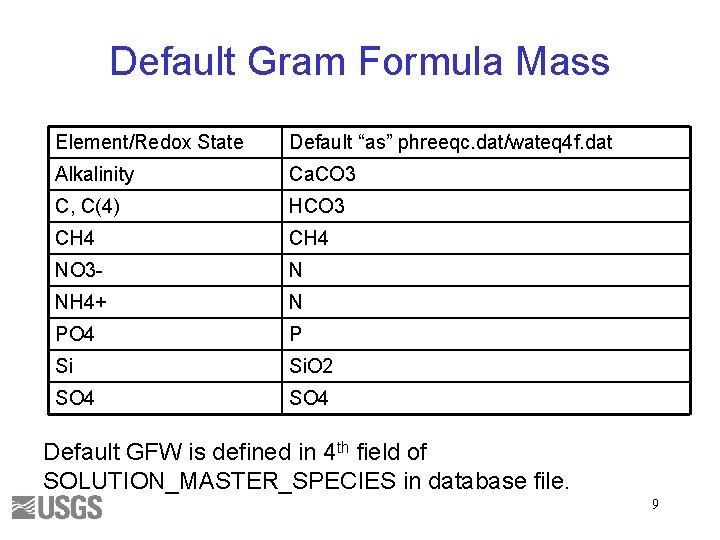
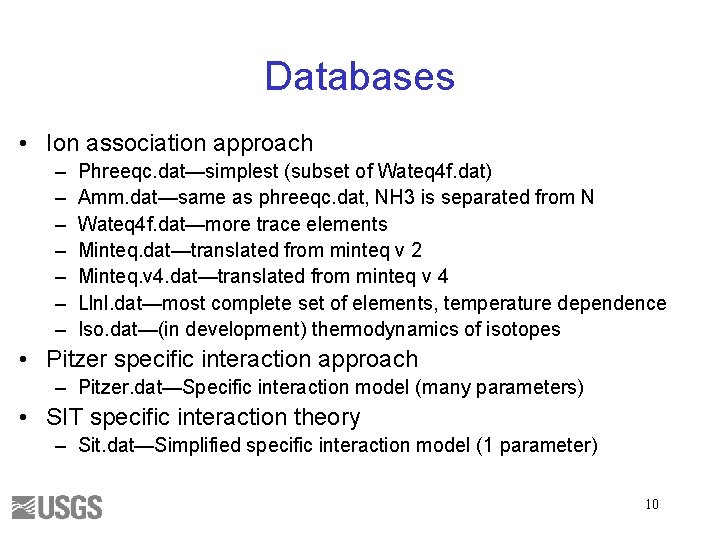
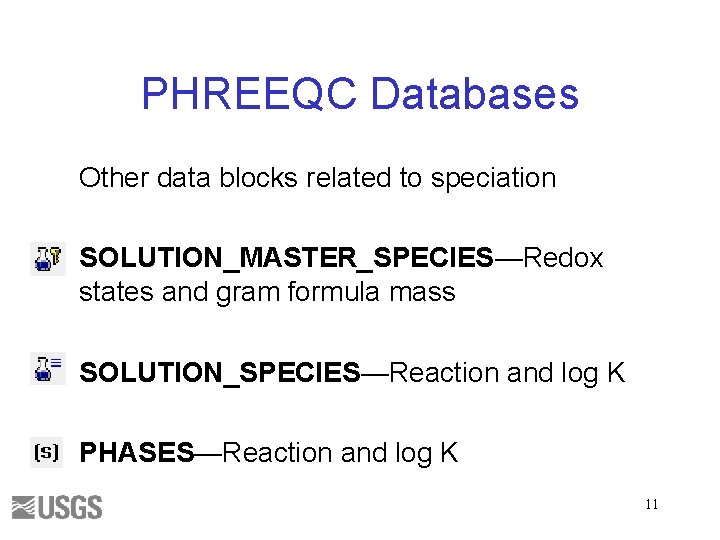
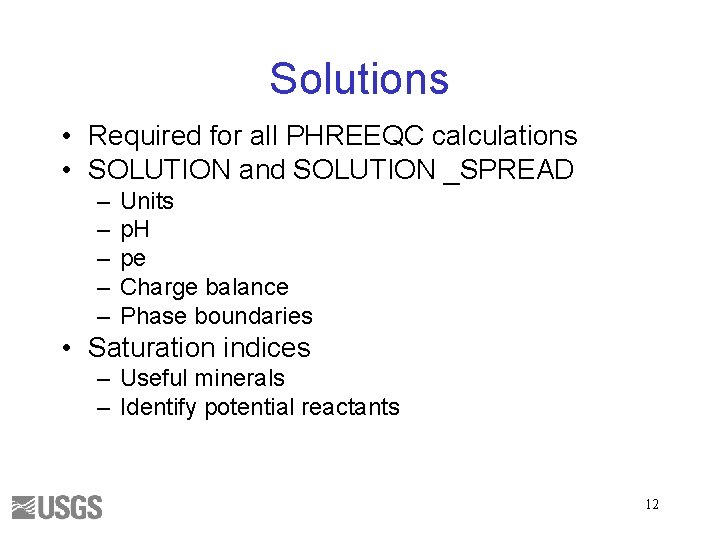
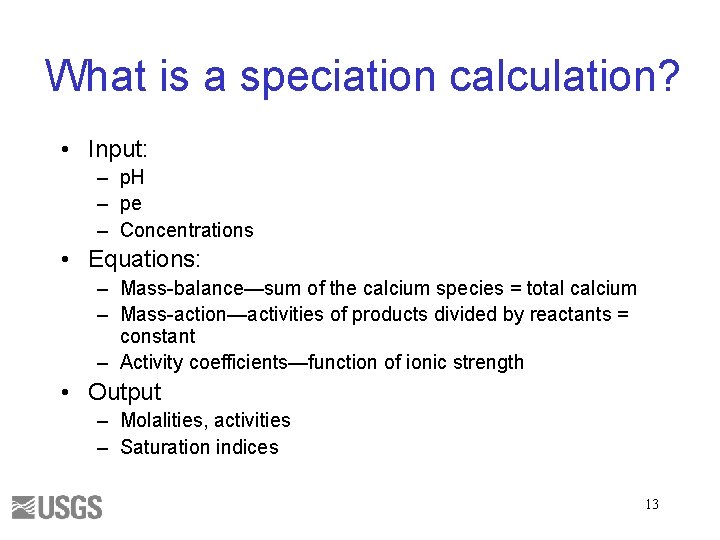
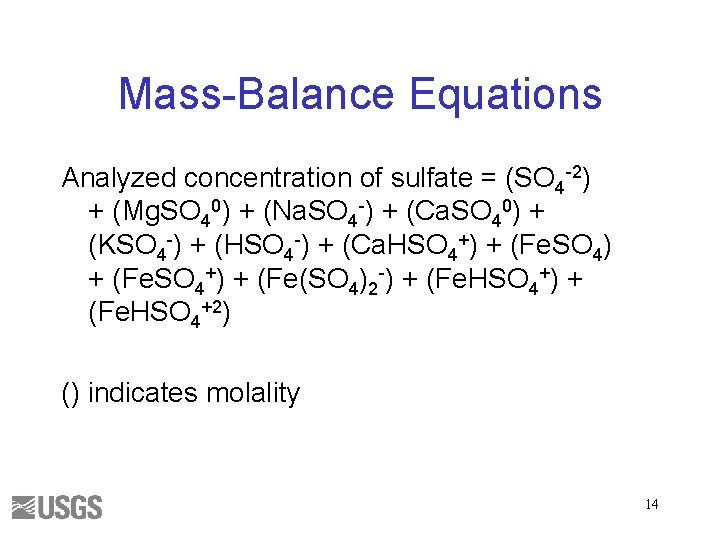
![Mass-Action Equations Ca+2 + SO 4 -2 = Ca. SO 40 [] indicates activity Mass-Action Equations Ca+2 + SO 4 -2 = Ca. SO 40 [] indicates activity](https://slidetodoc.com/presentation_image/200b8de3ad78ea91e8cd99b28236b61f/image-15.jpg)
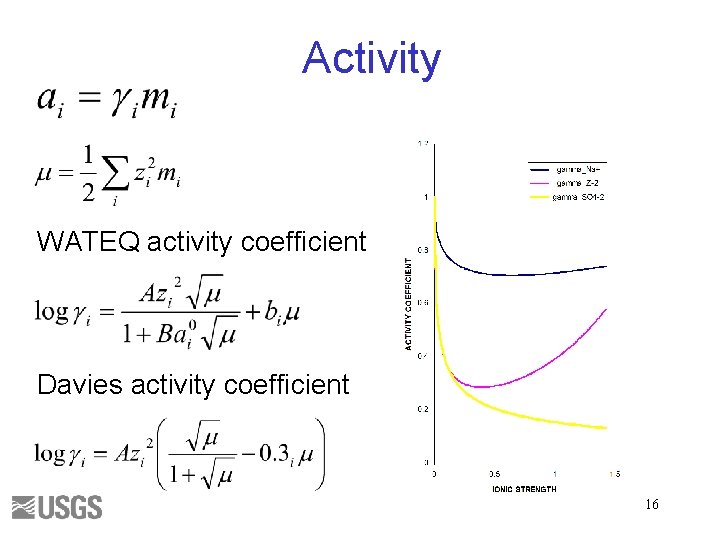
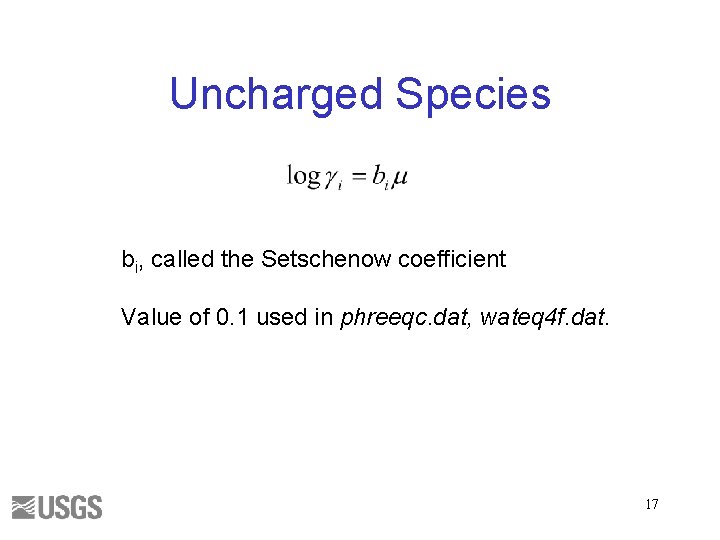
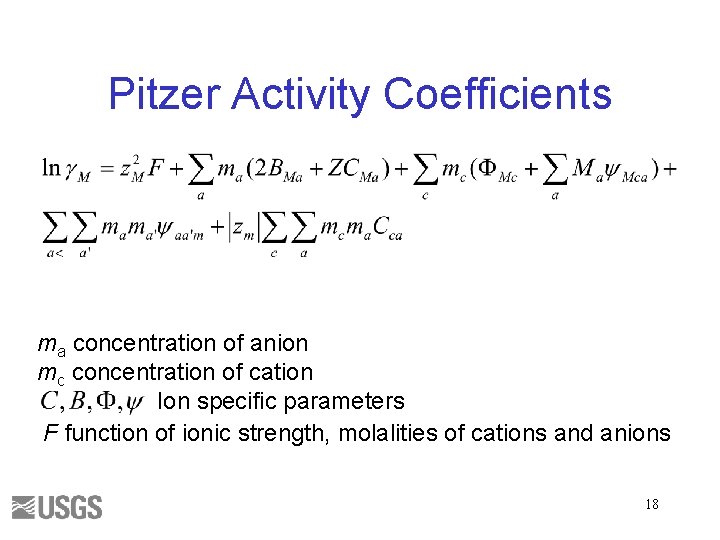
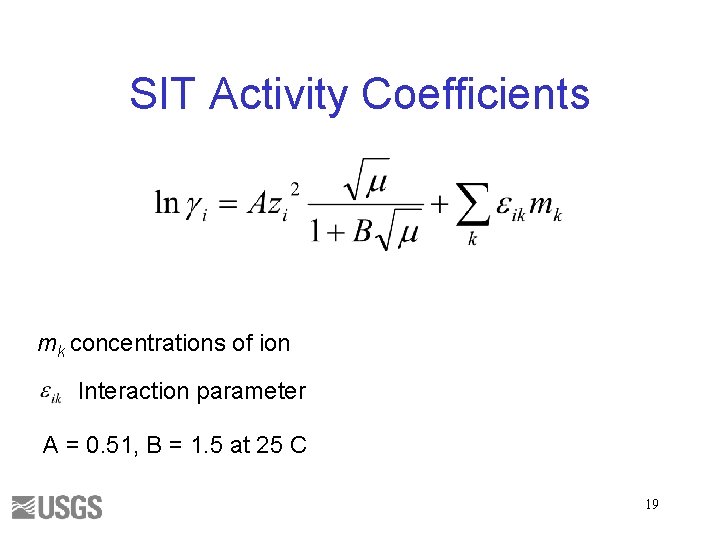
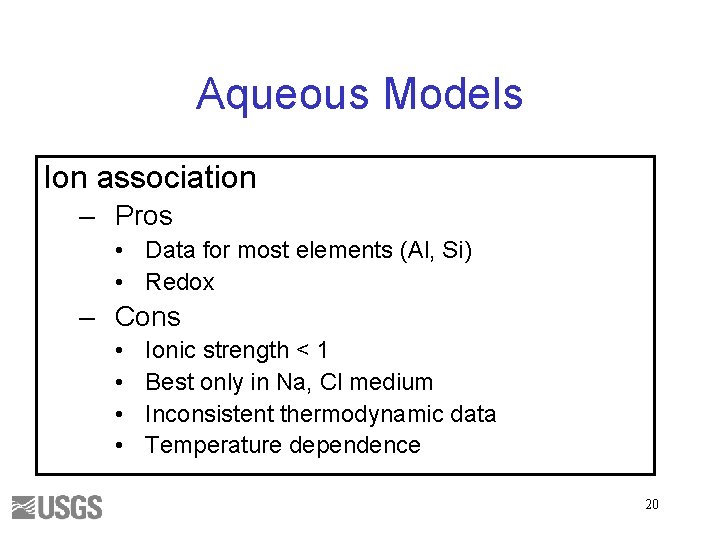
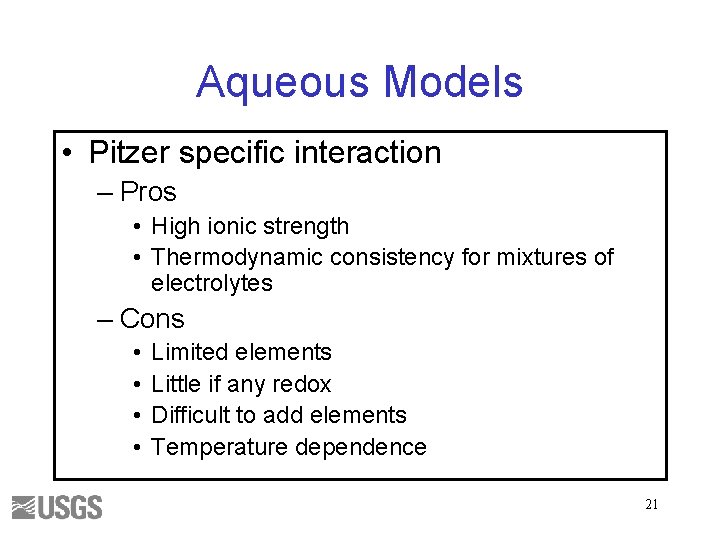
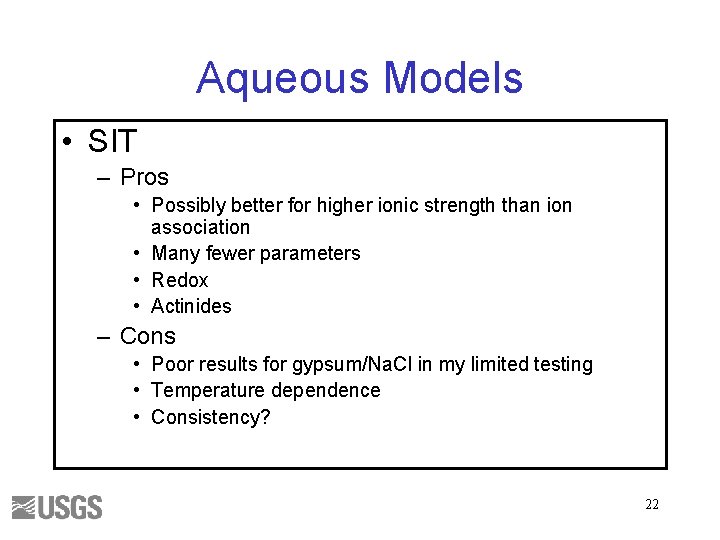
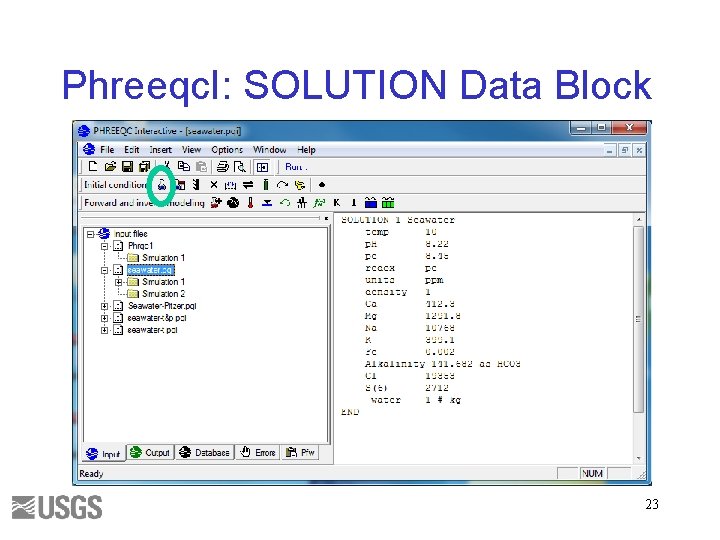
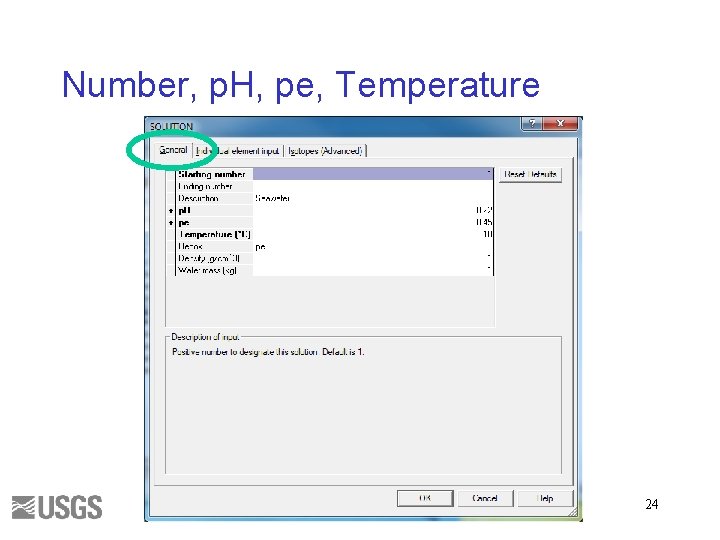
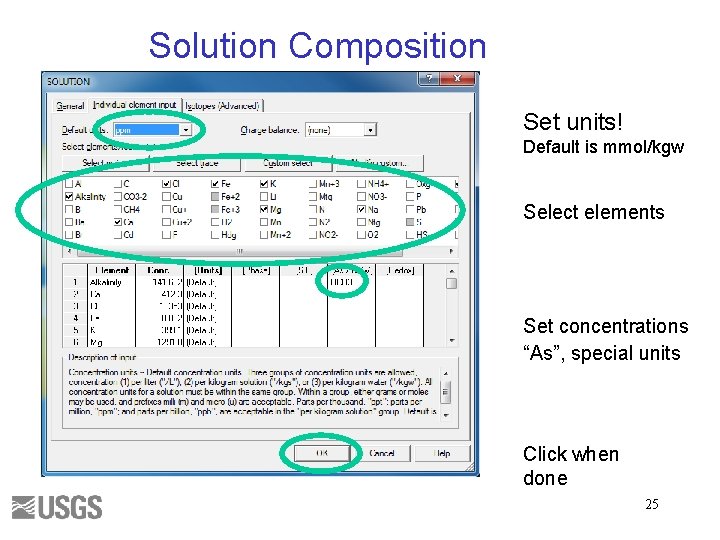
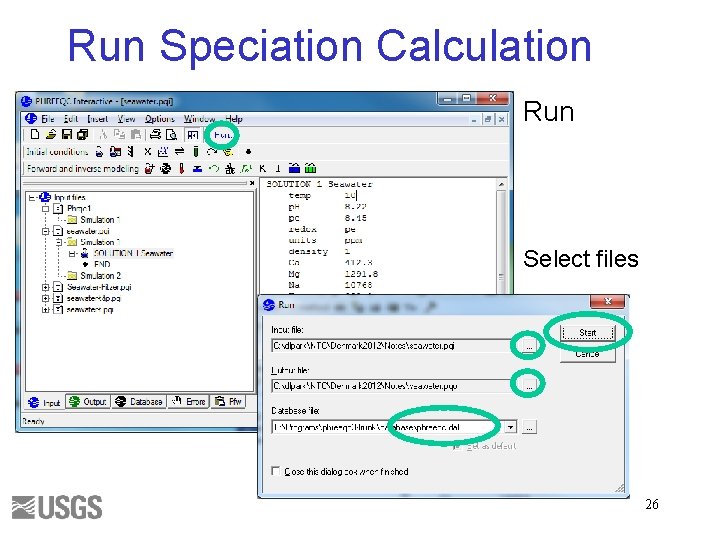
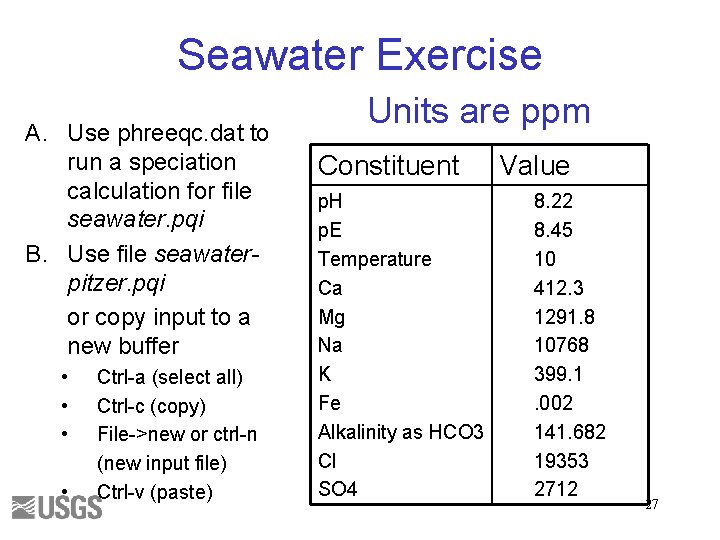
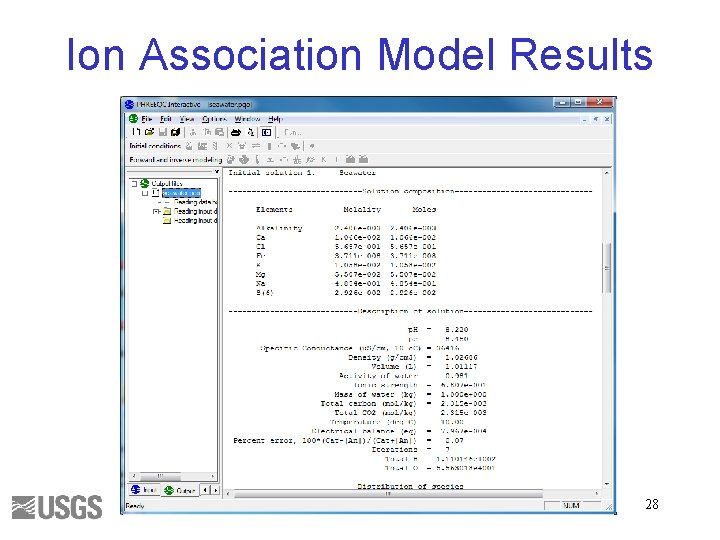
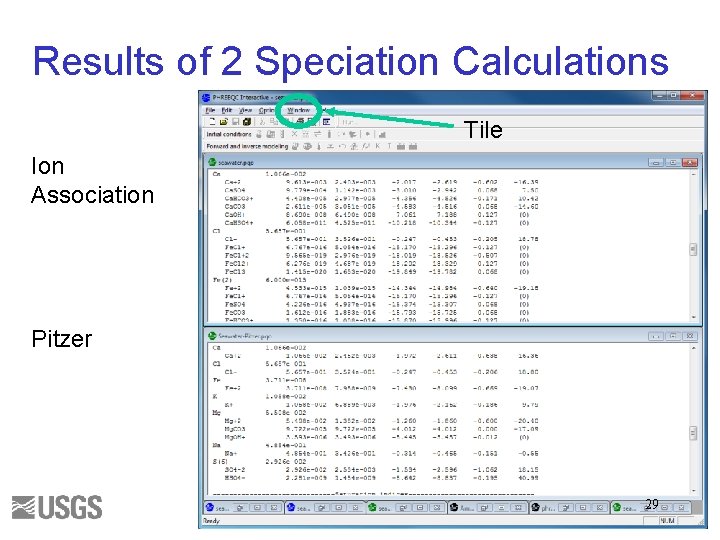
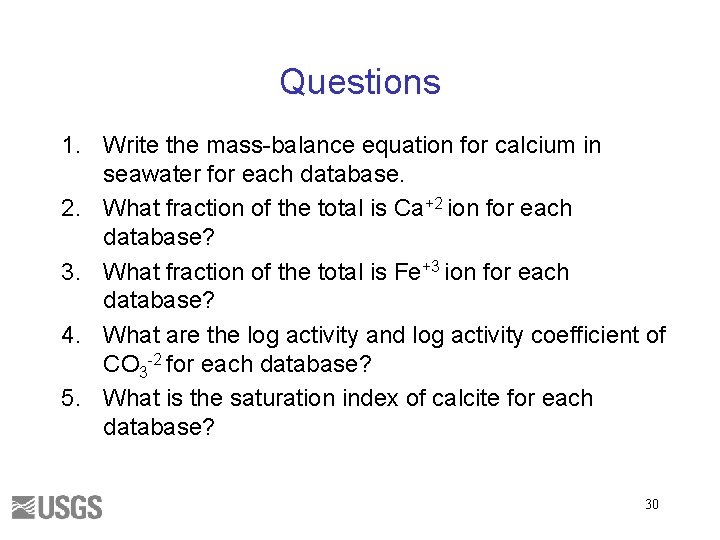
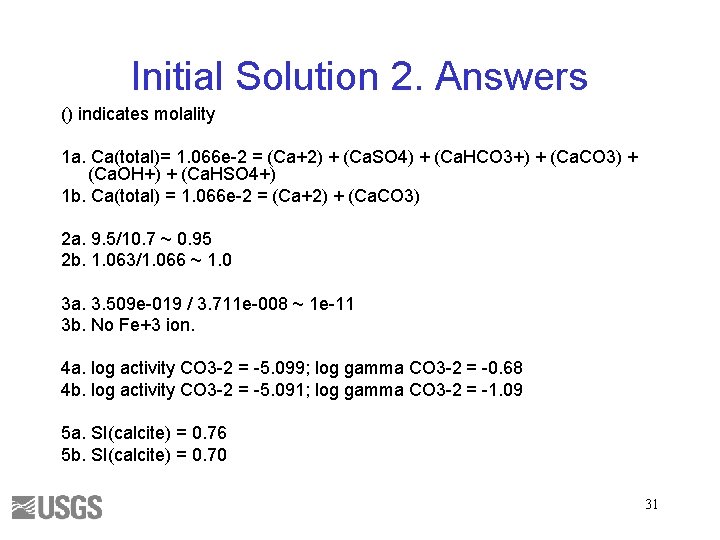
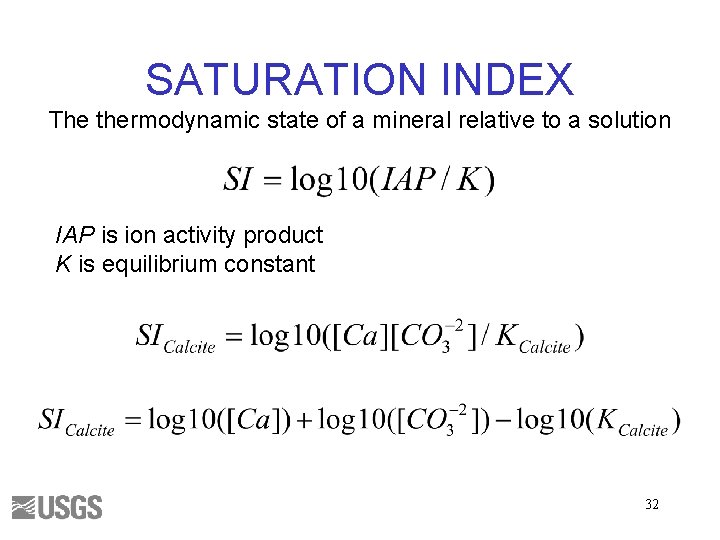
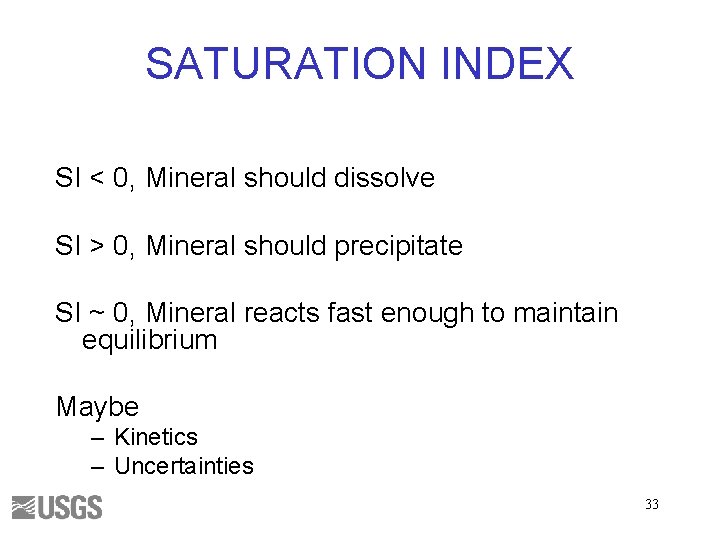
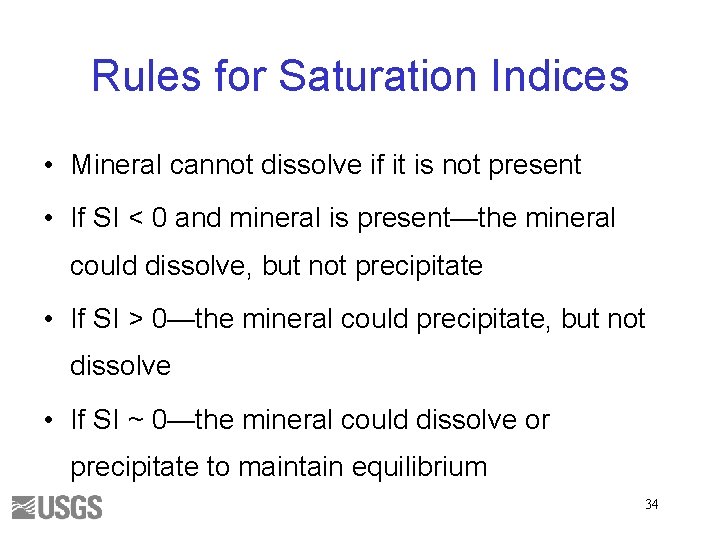
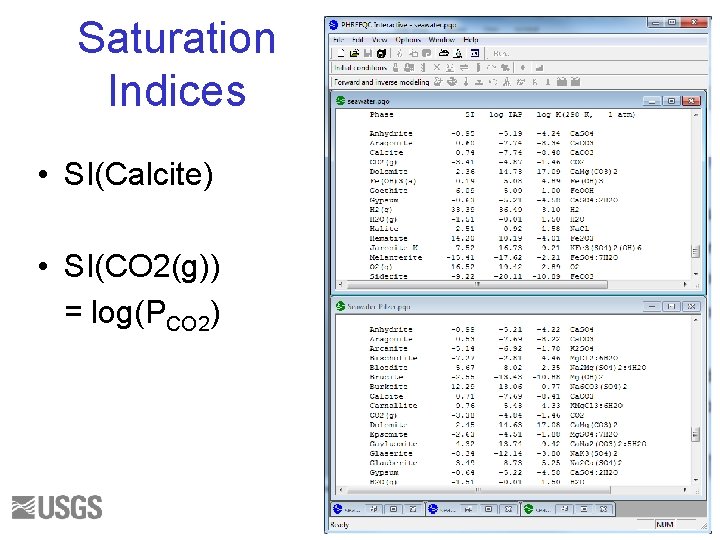
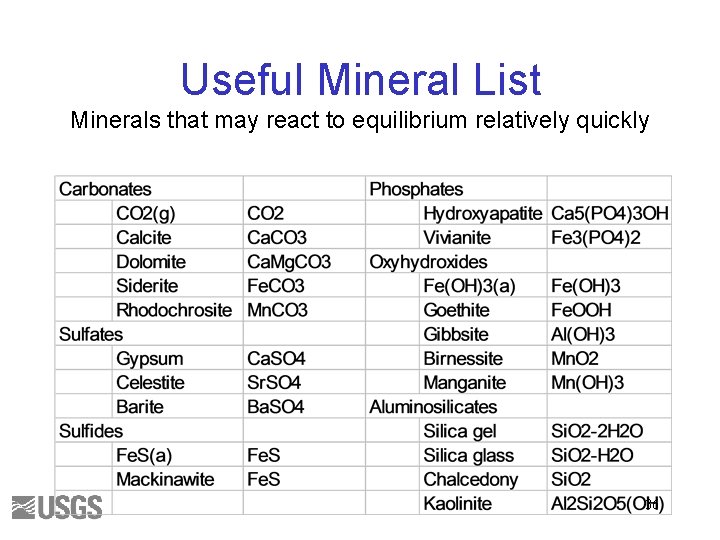
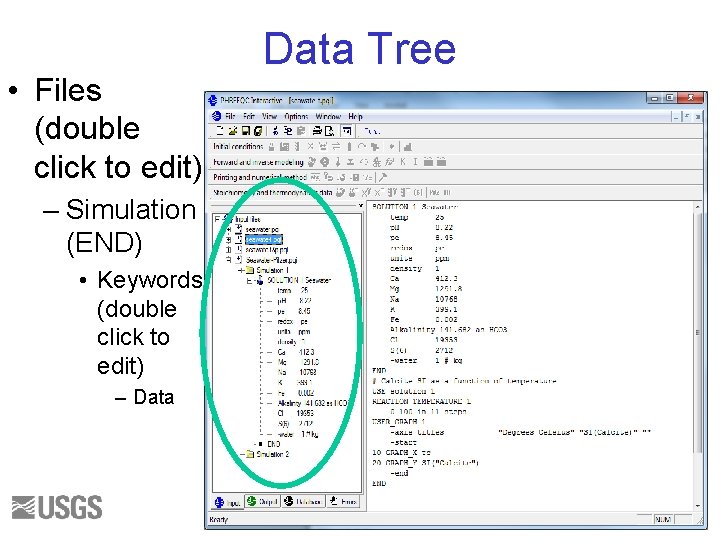
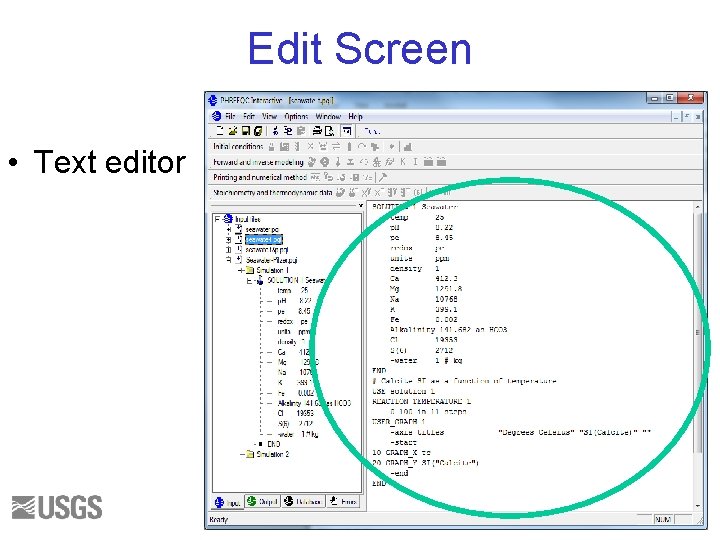
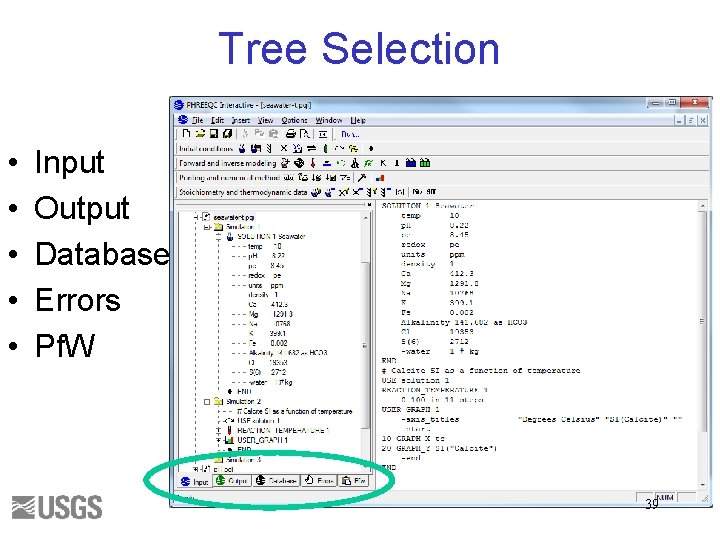
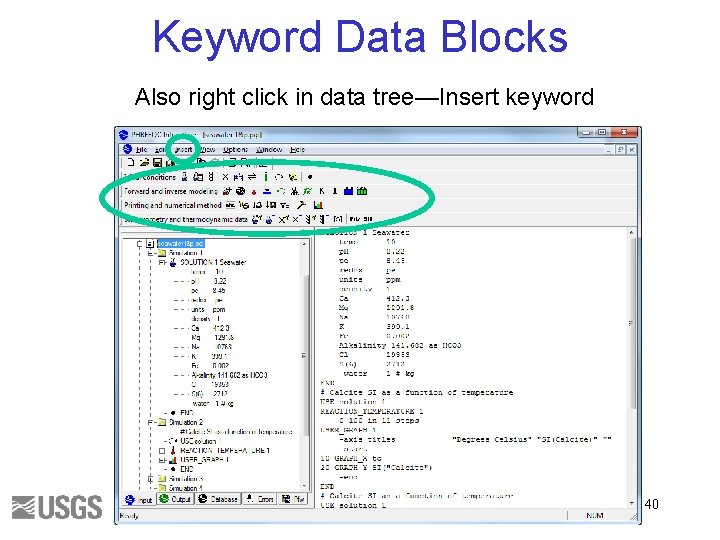
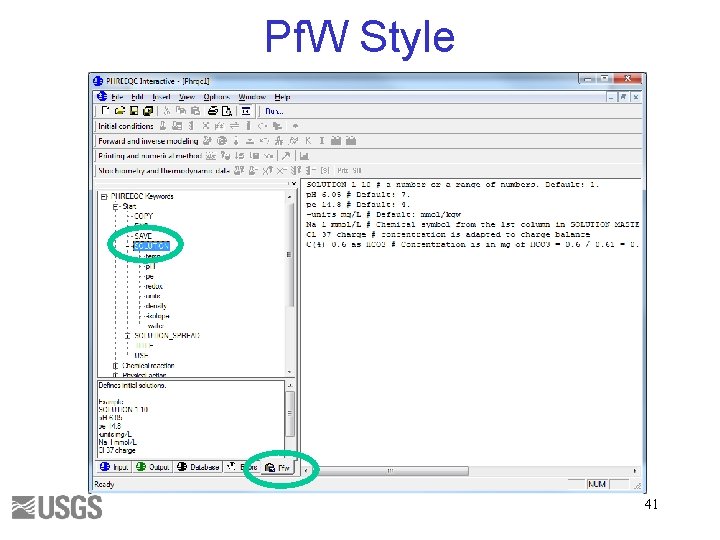
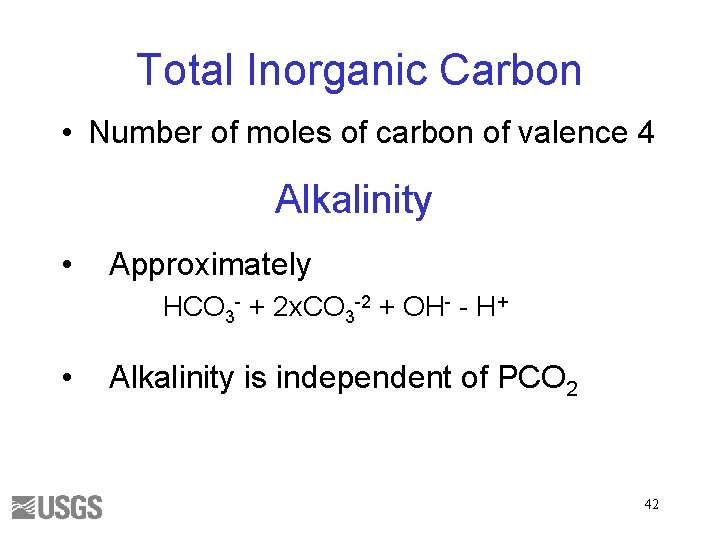
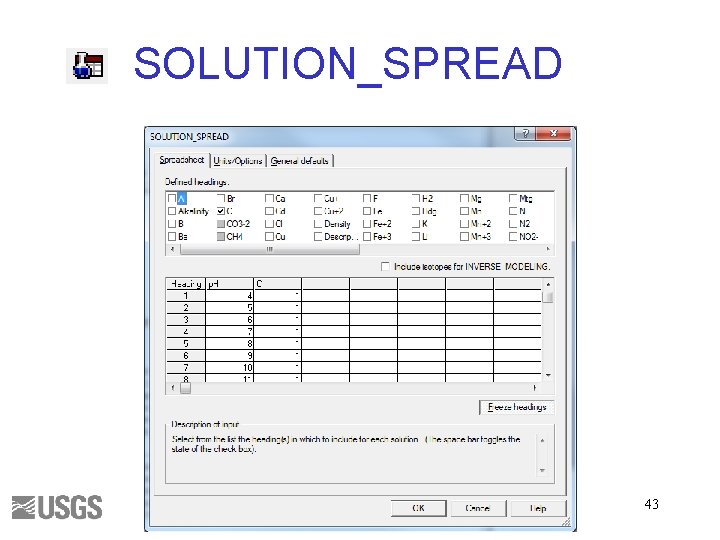
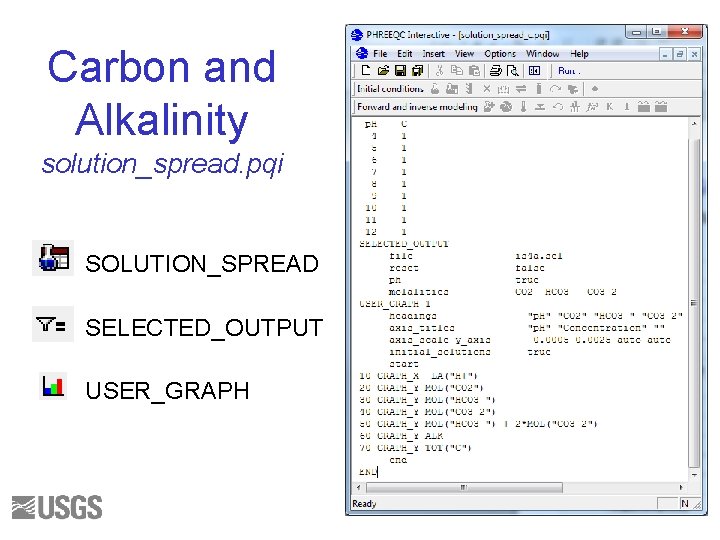
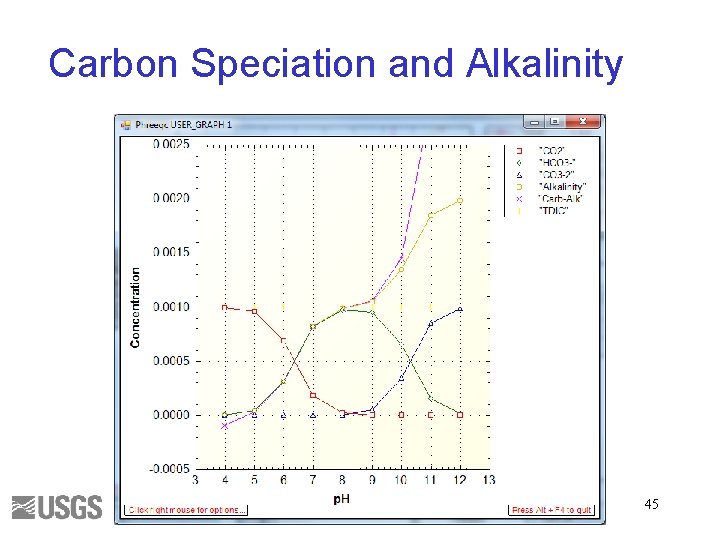
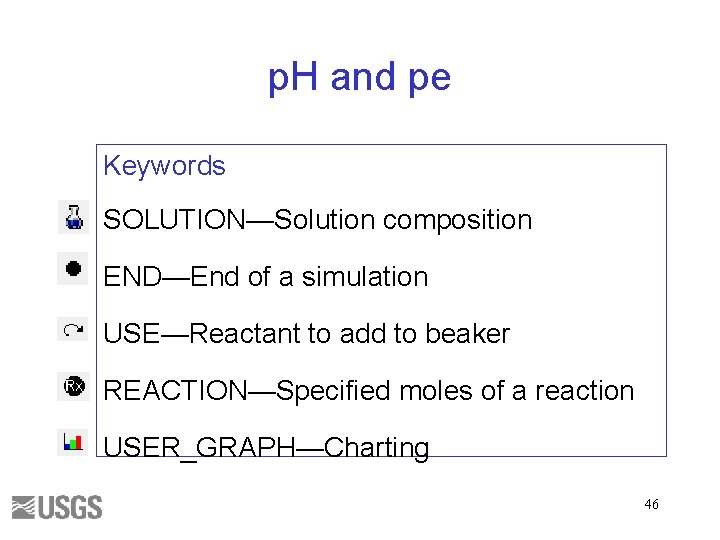
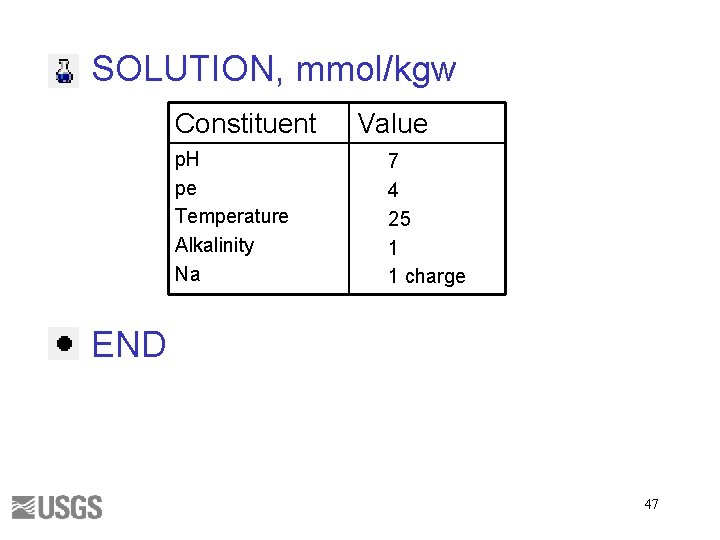
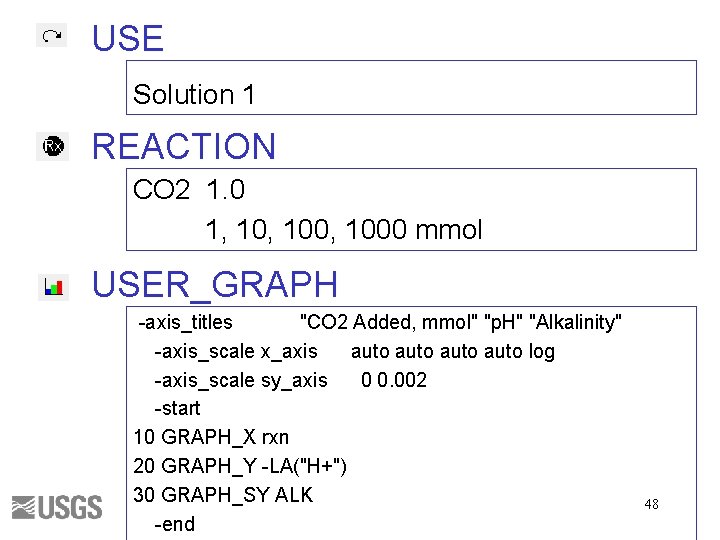
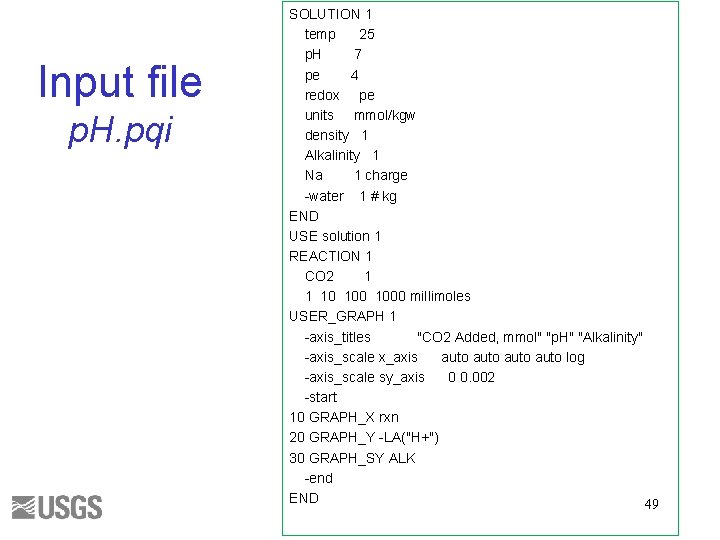
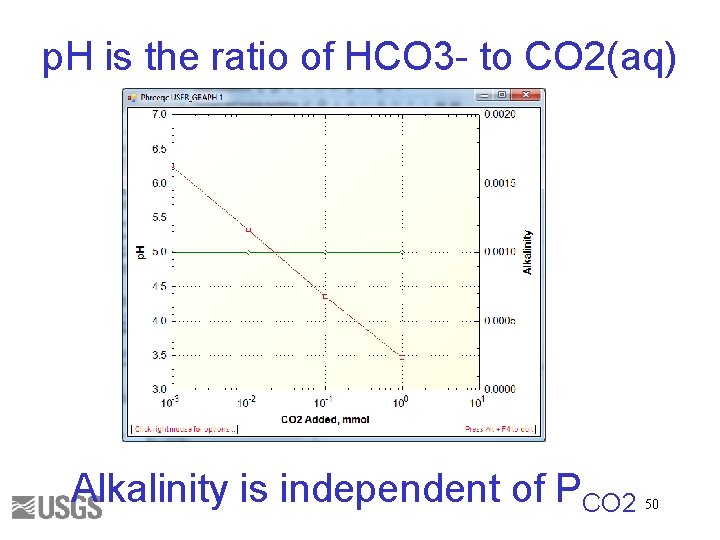
![What is p. H? p. H = 6. 3 + log[(HCO 3 -)/(CO 2)] What is p. H? p. H = 6. 3 + log[(HCO 3 -)/(CO 2)]](https://slidetodoc.com/presentation_image/200b8de3ad78ea91e8cd99b28236b61f/image-51.jpg)
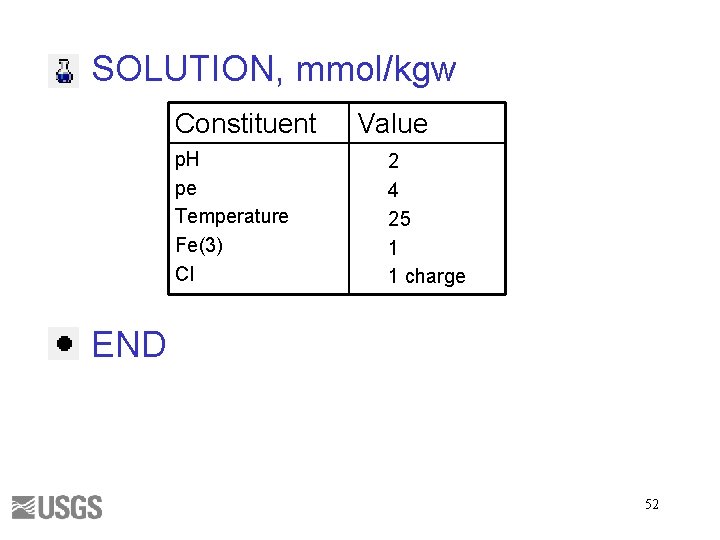
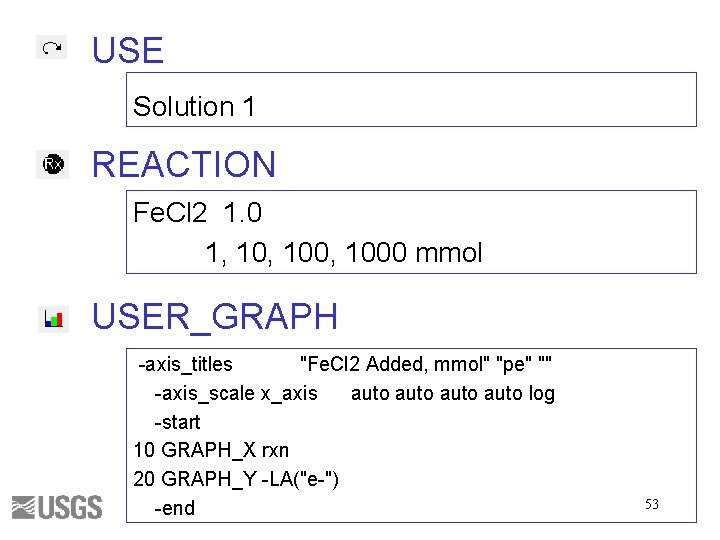
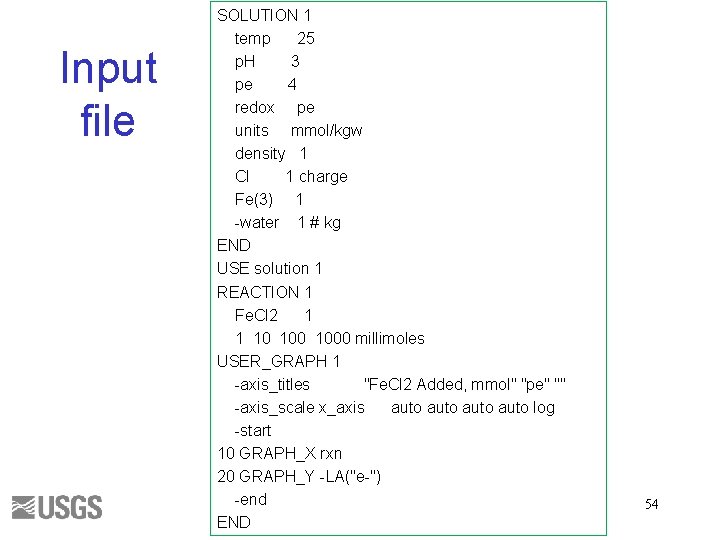
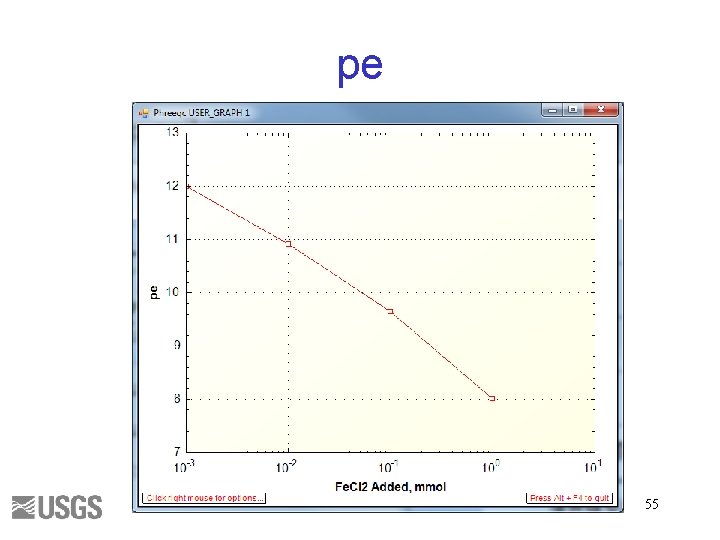
![What is pe? Fe+2 = Fe+3 + epe = log( [Fe+3]/[Fe+2] ) + 13 What is pe? Fe+2 = Fe+3 + epe = log( [Fe+3]/[Fe+2] ) + 13](https://slidetodoc.com/presentation_image/200b8de3ad78ea91e8cd99b28236b61f/image-56.jpg)
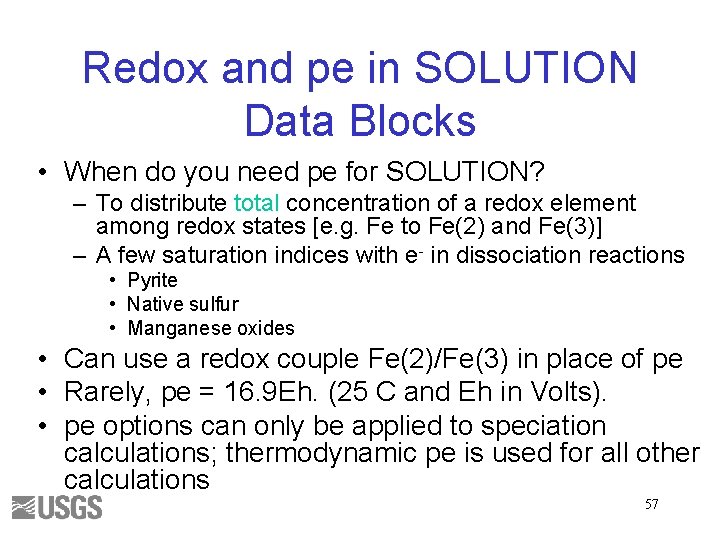
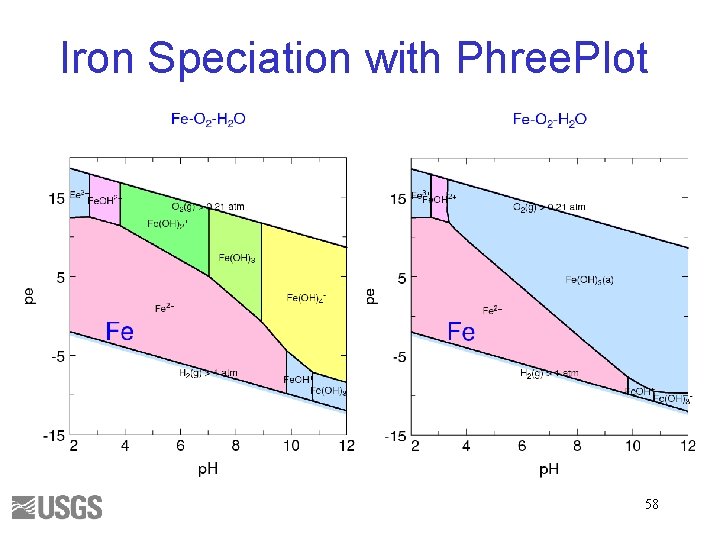
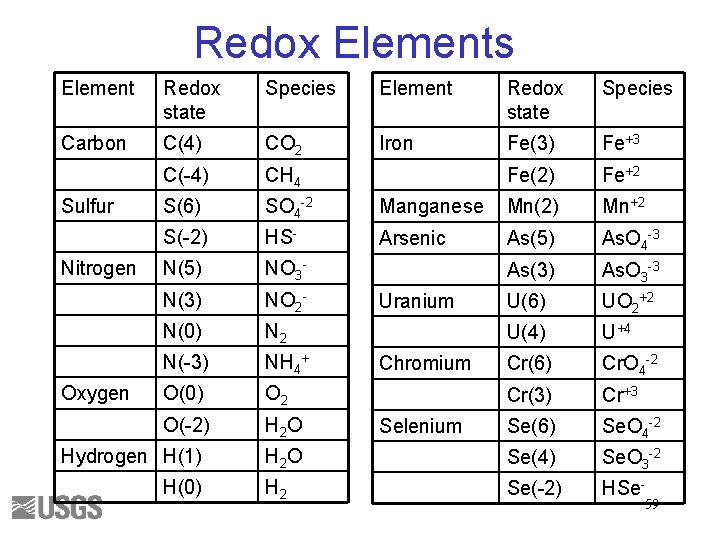
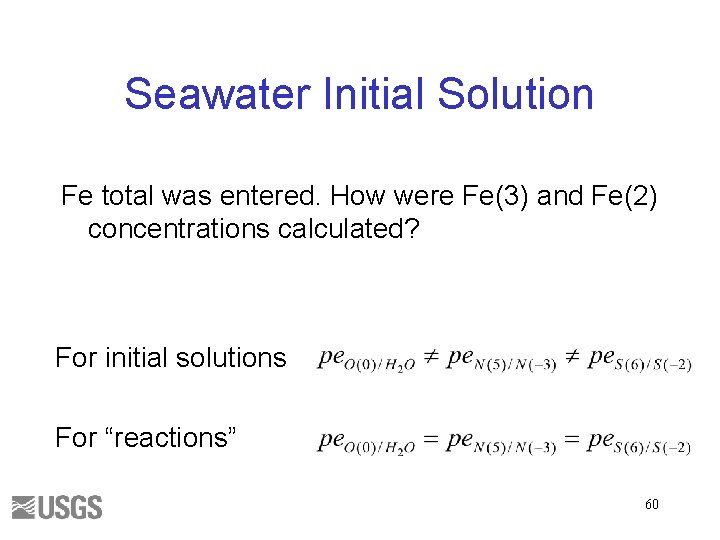
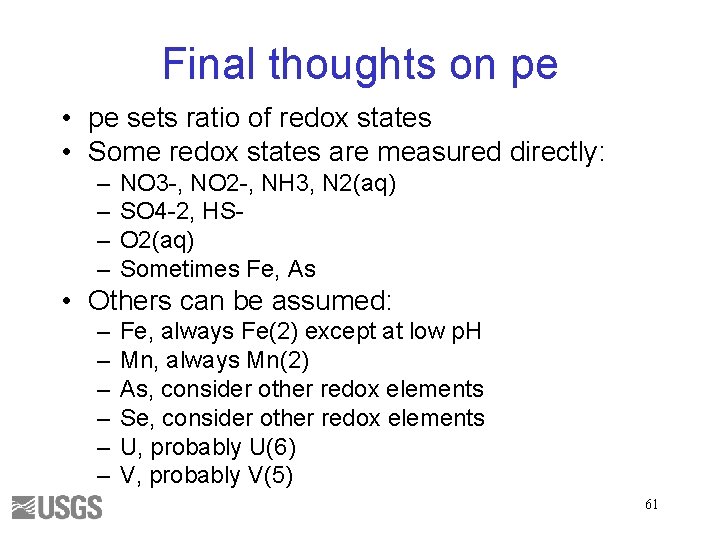
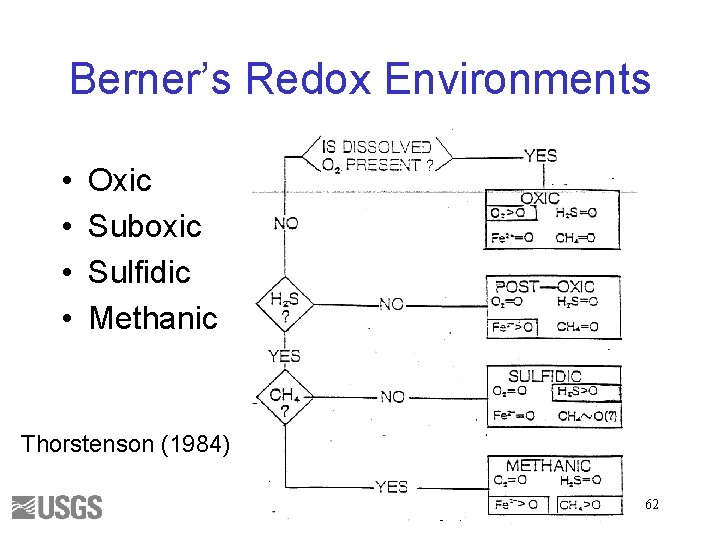
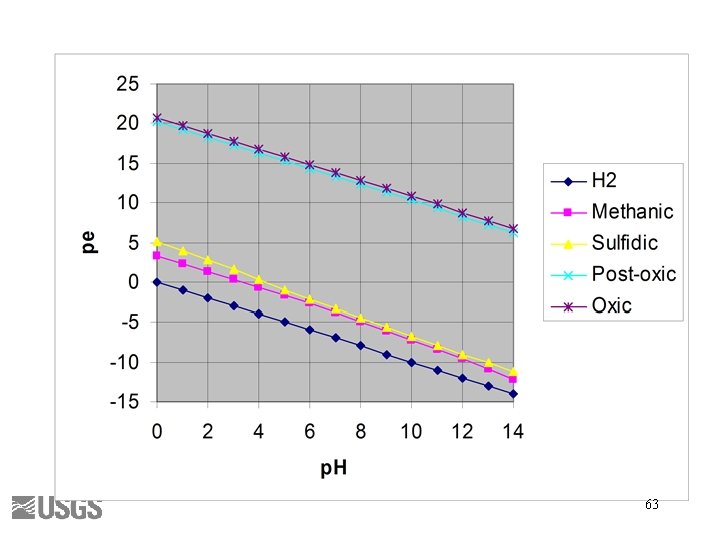
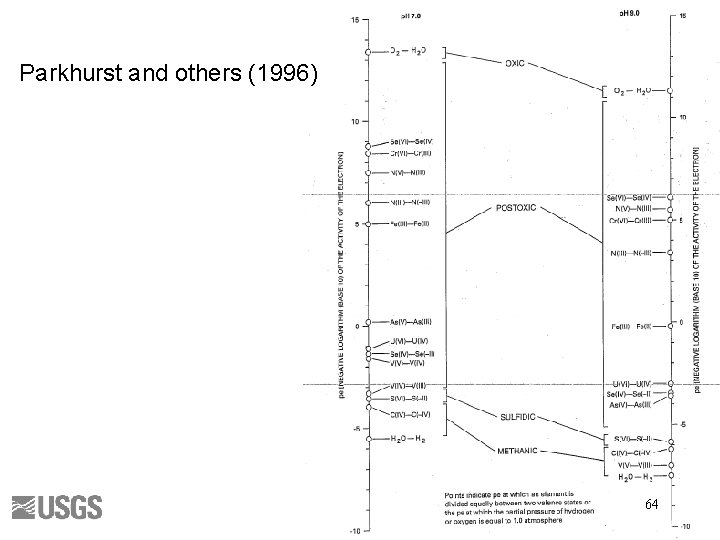
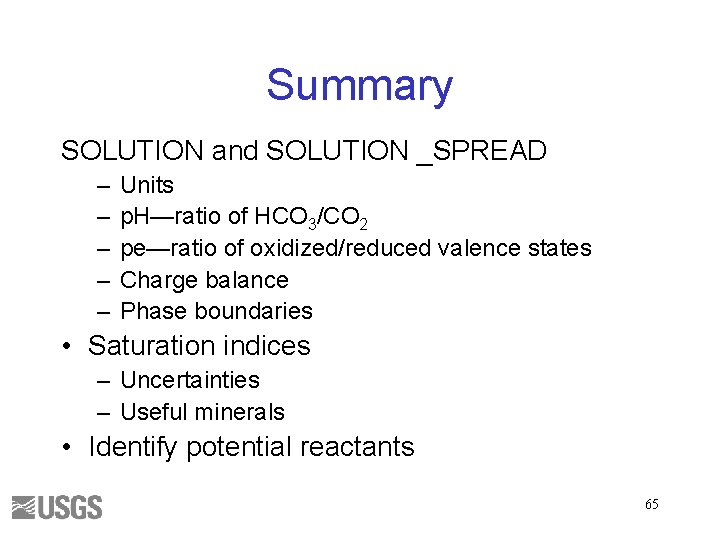
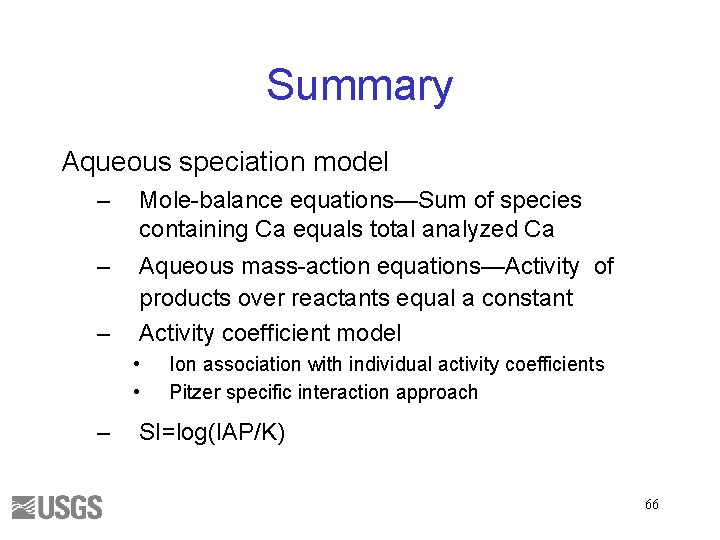
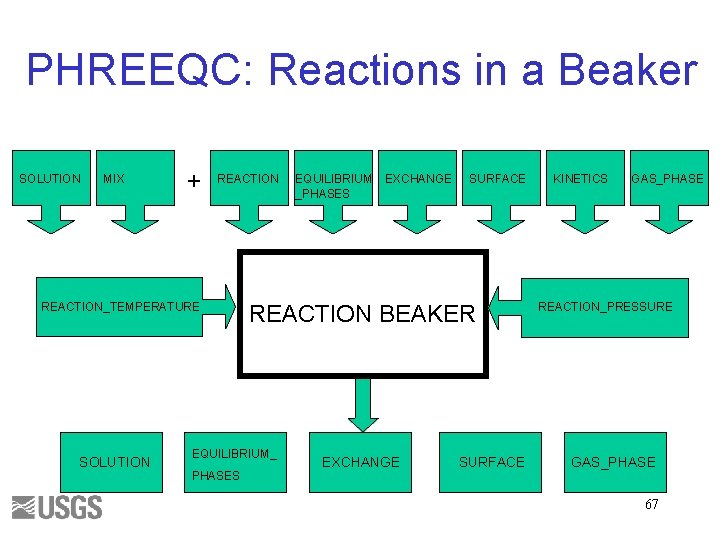
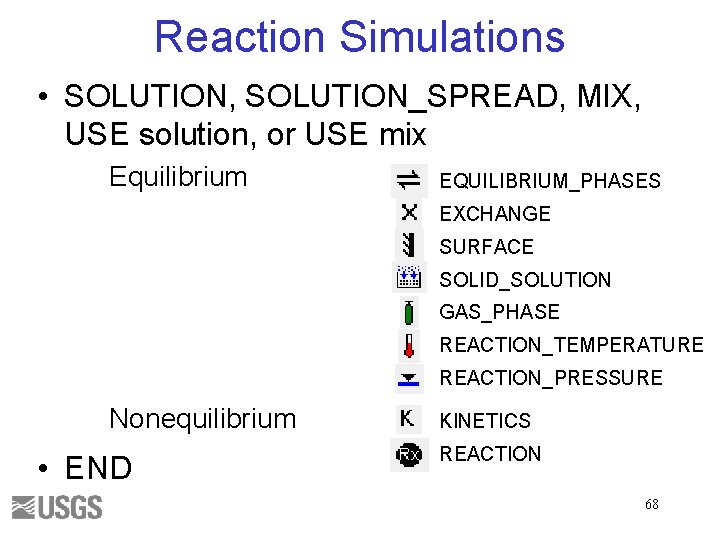
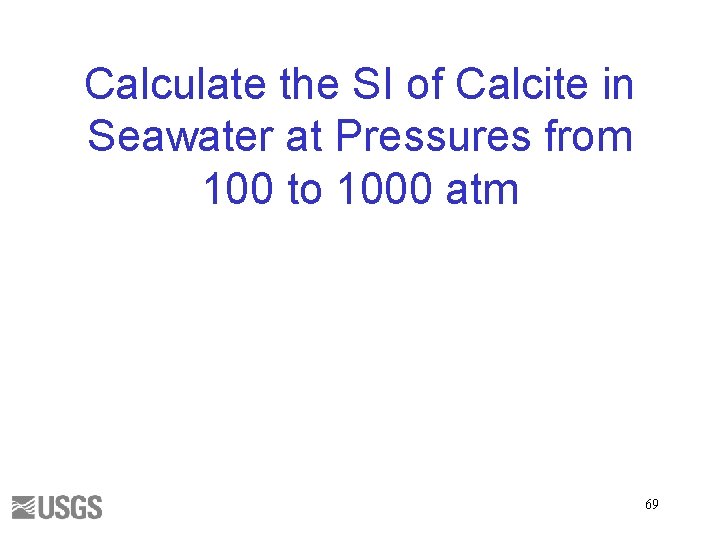
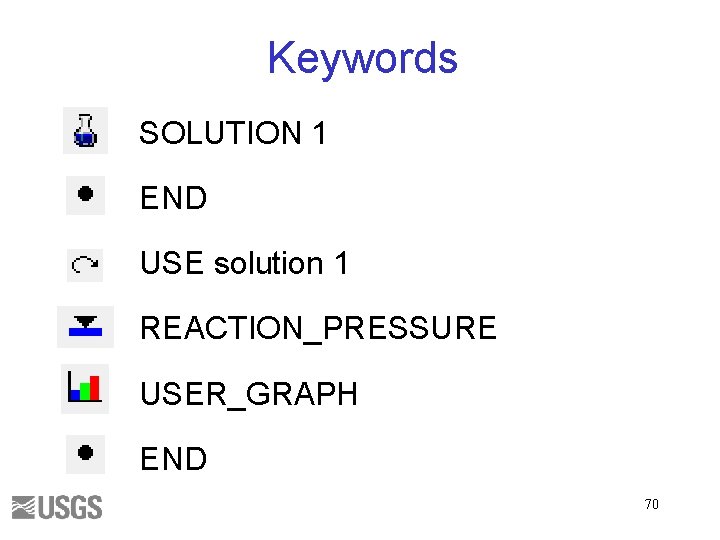
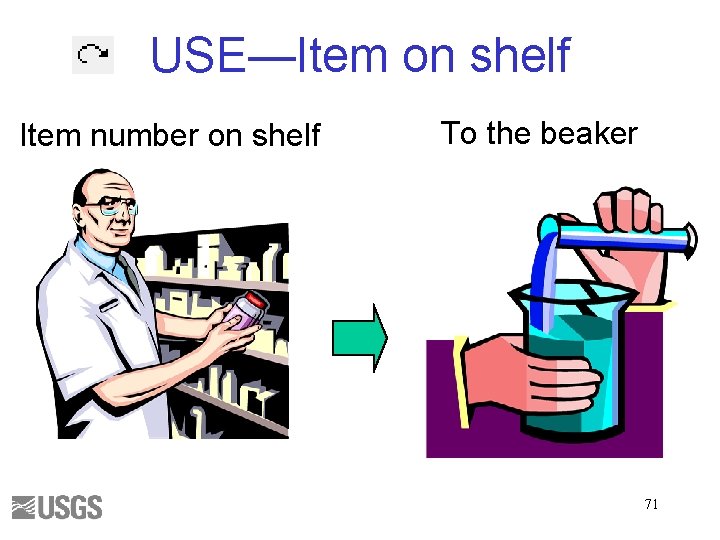
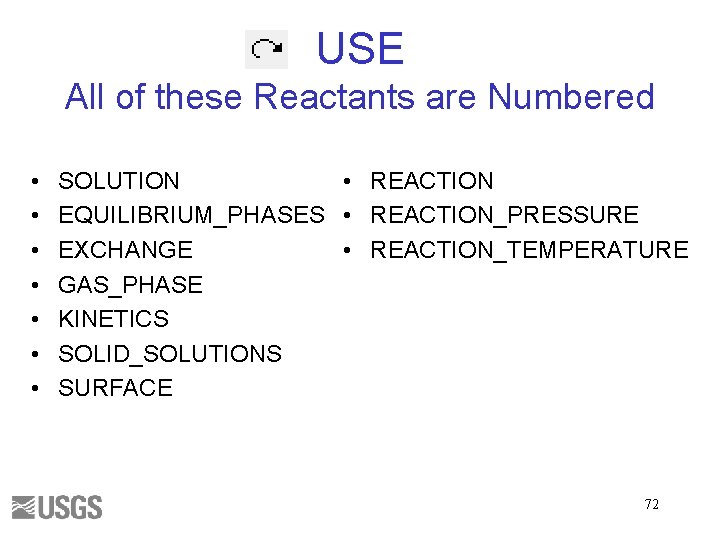
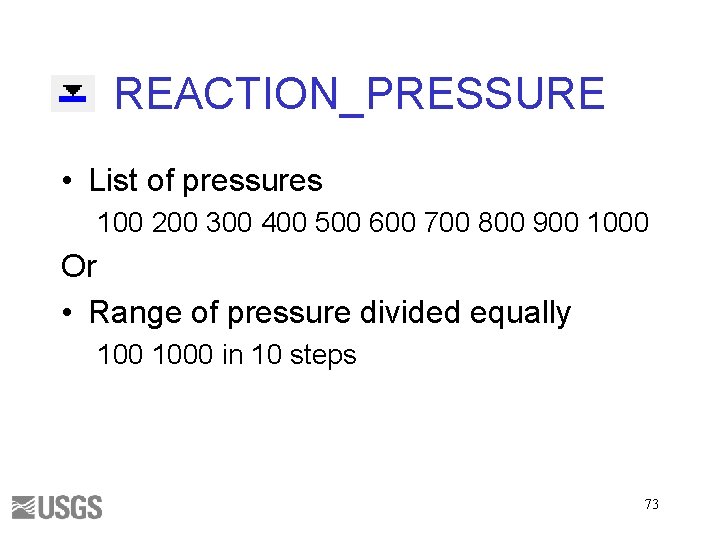
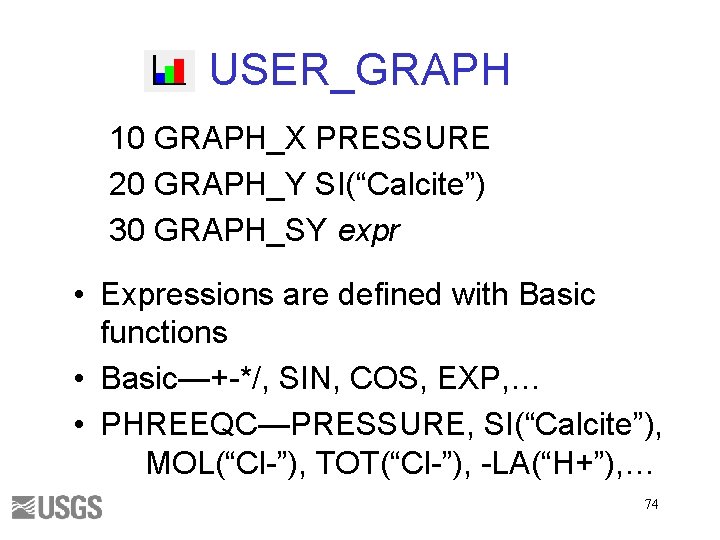
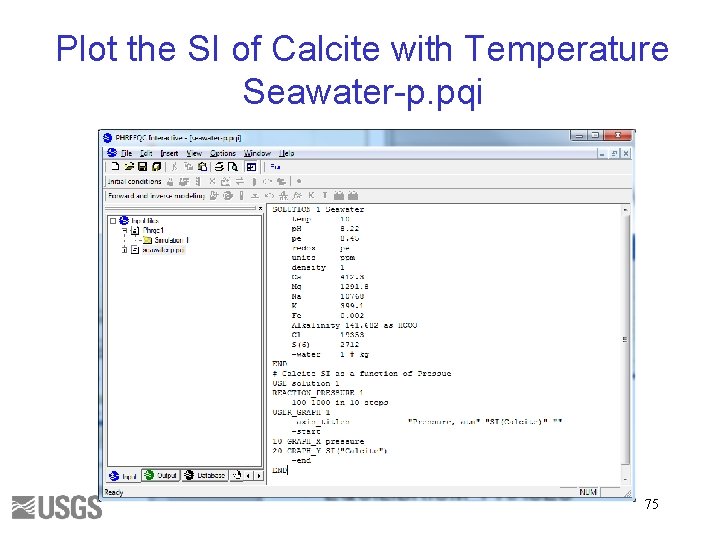
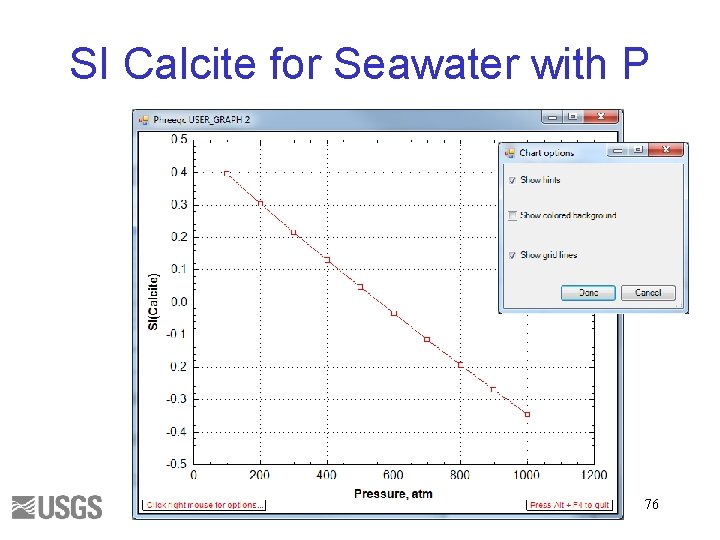
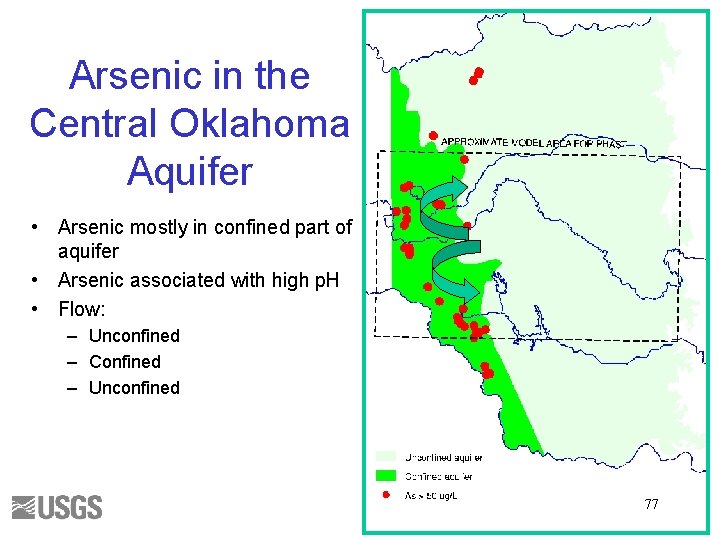
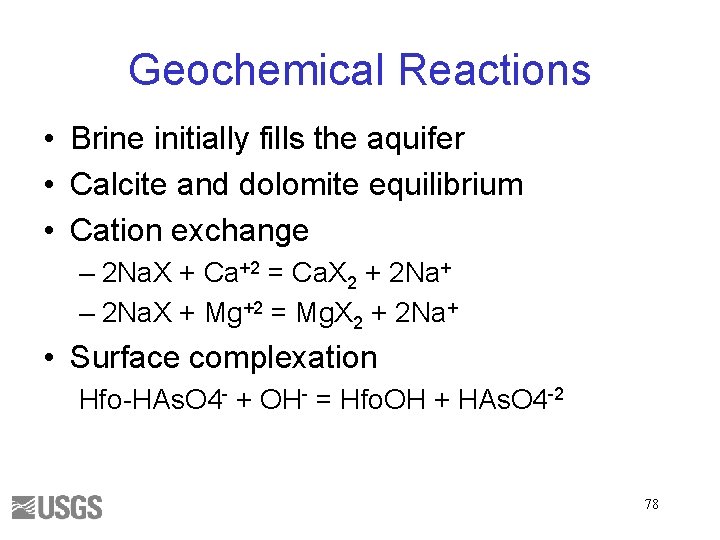
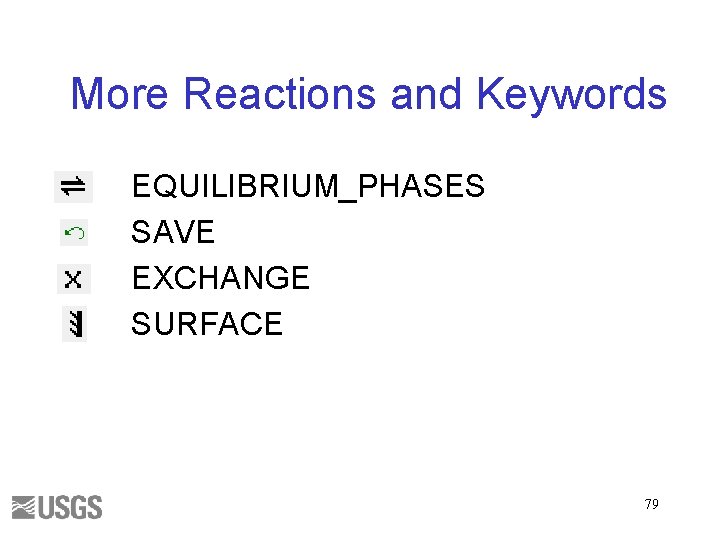
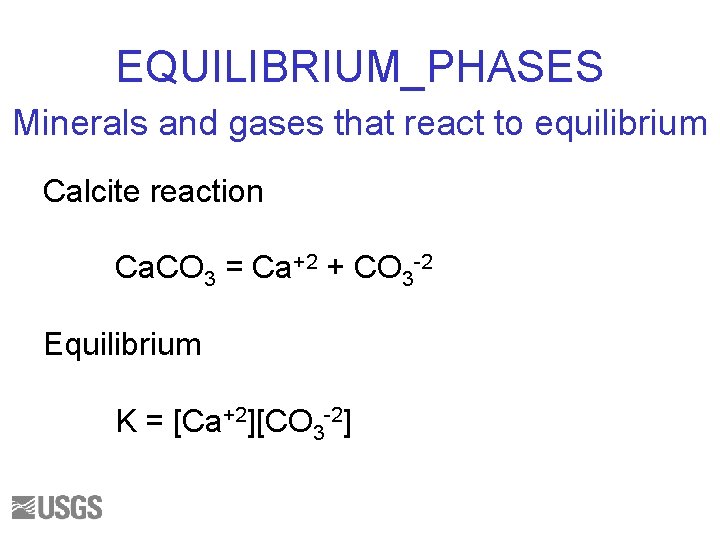
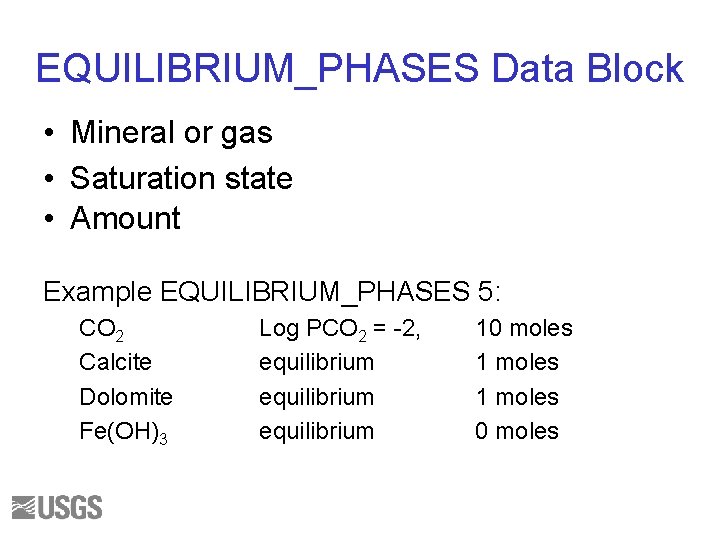
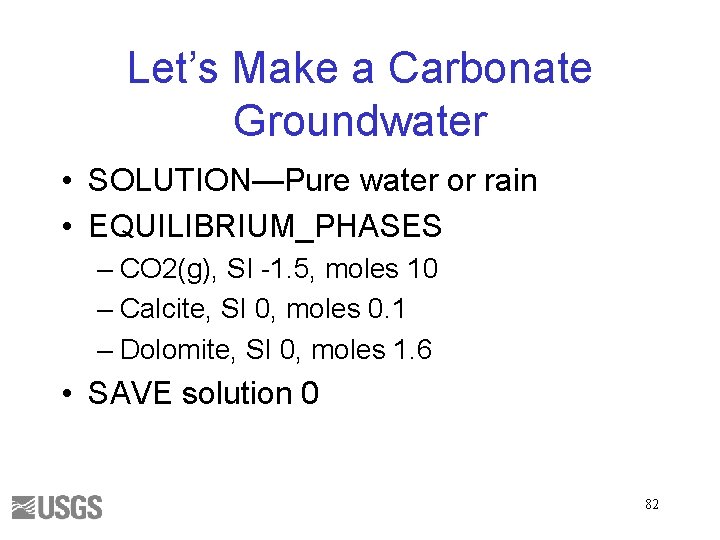
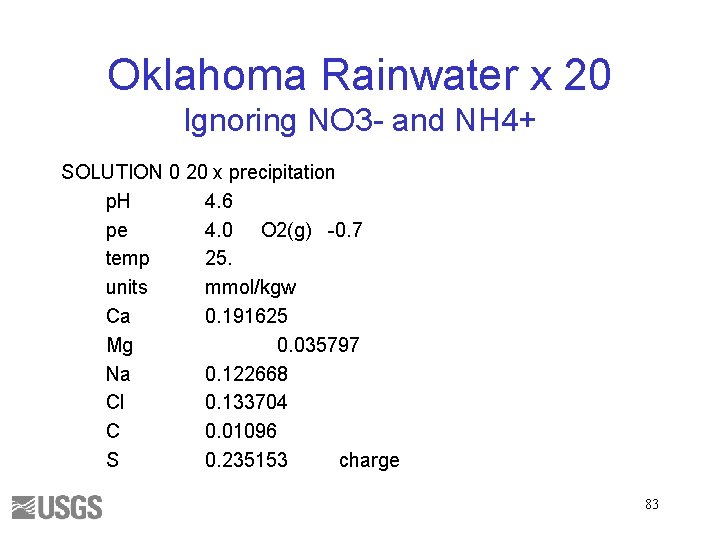
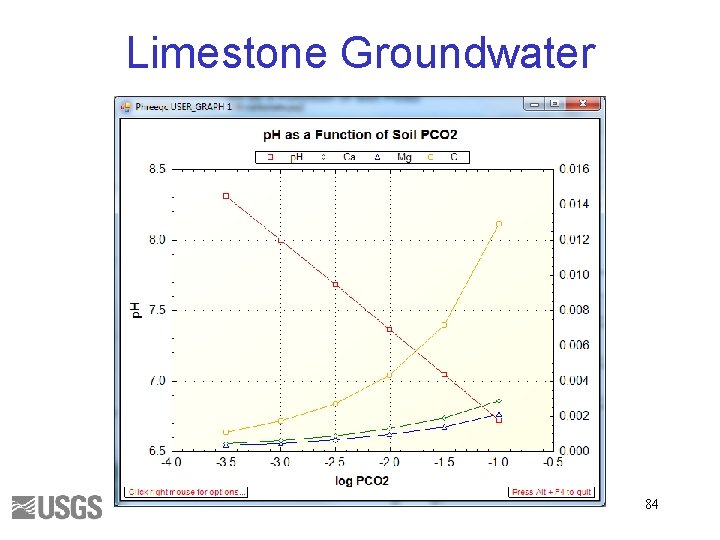
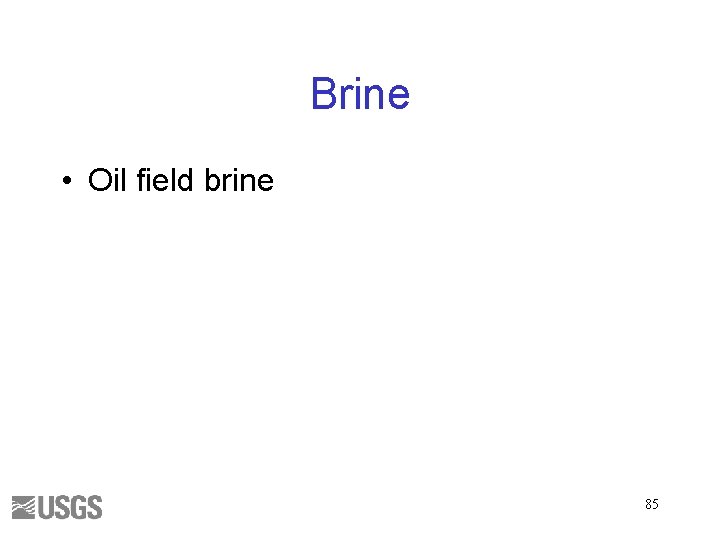
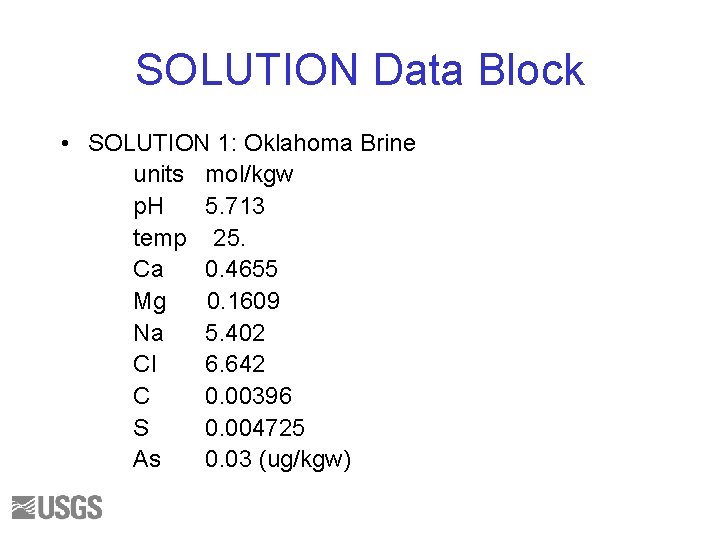
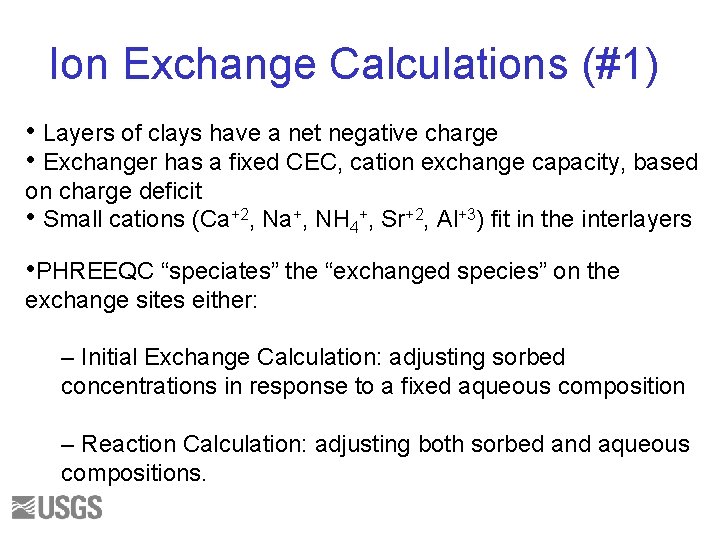
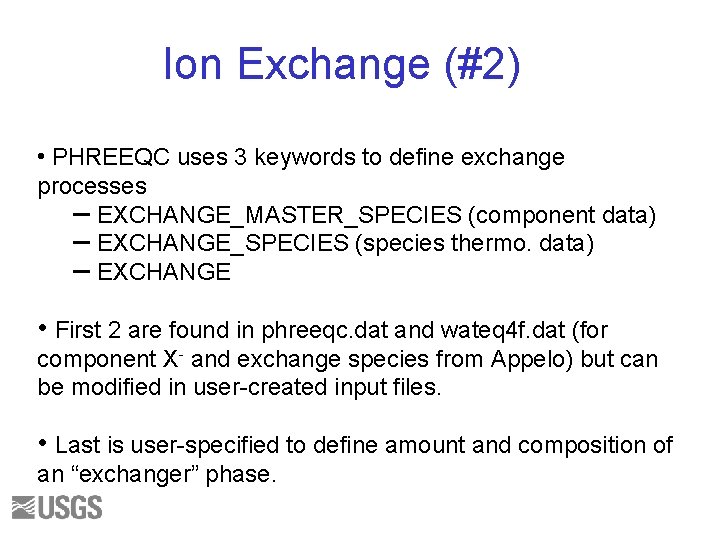
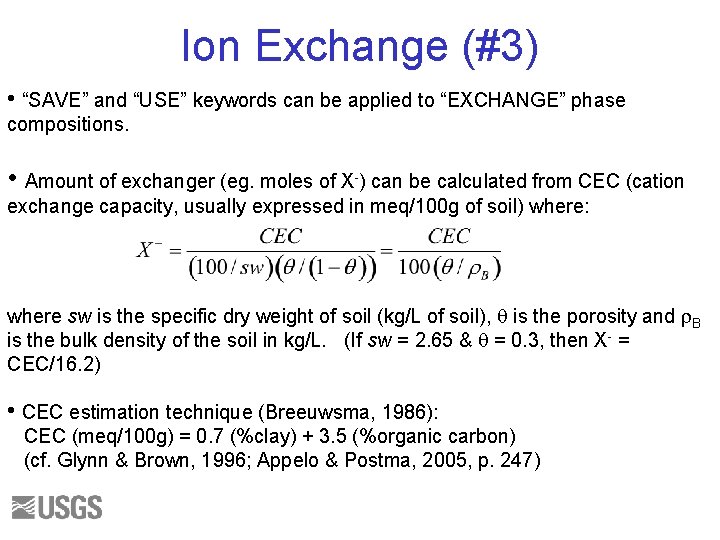
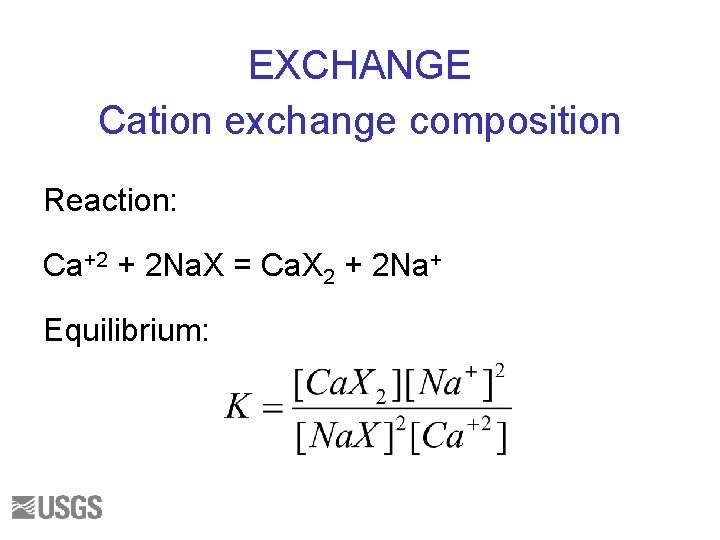
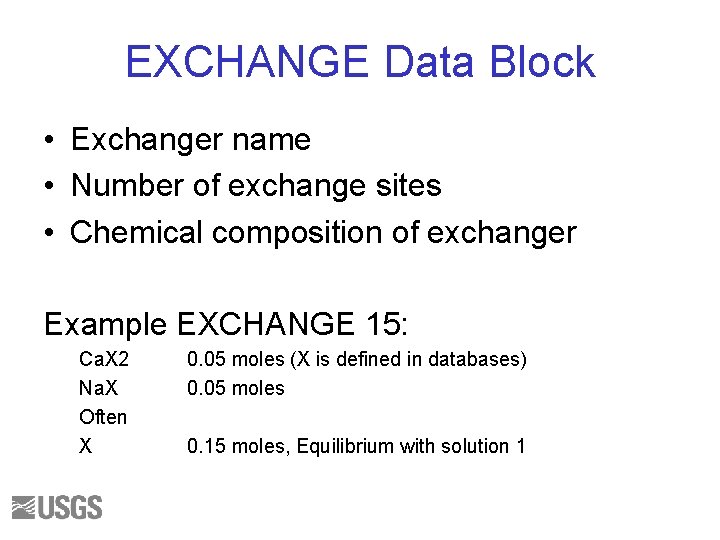
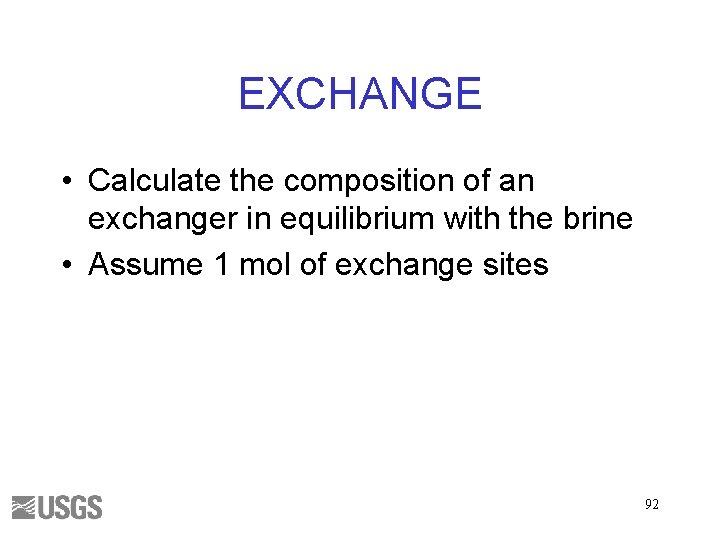
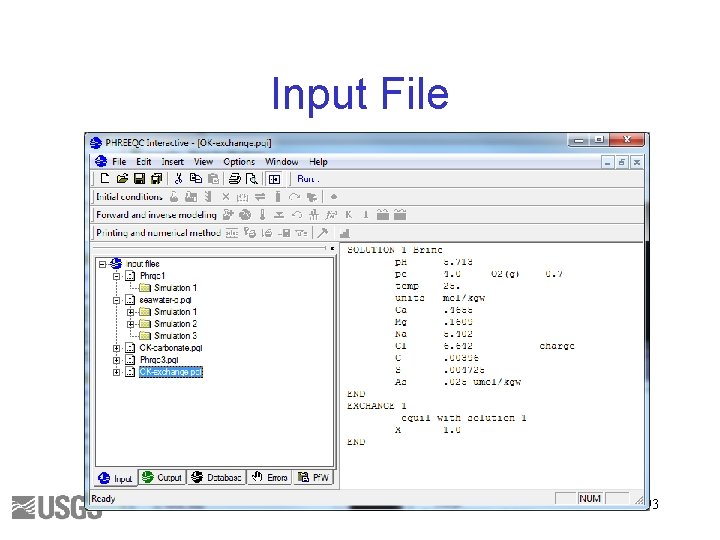
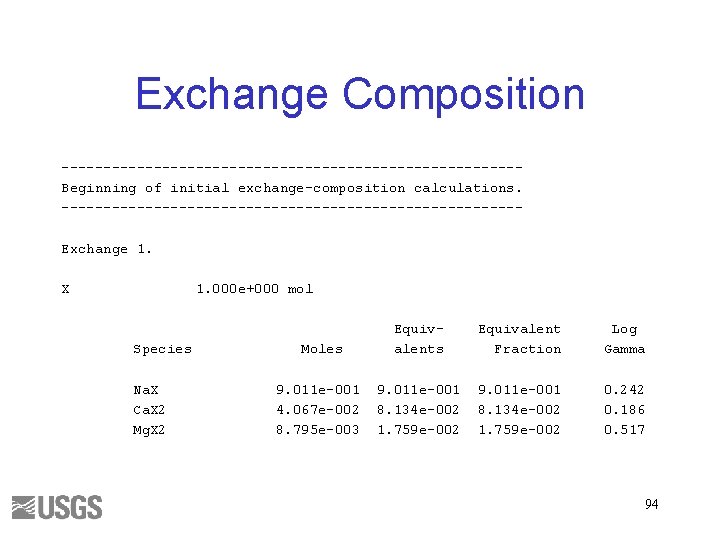
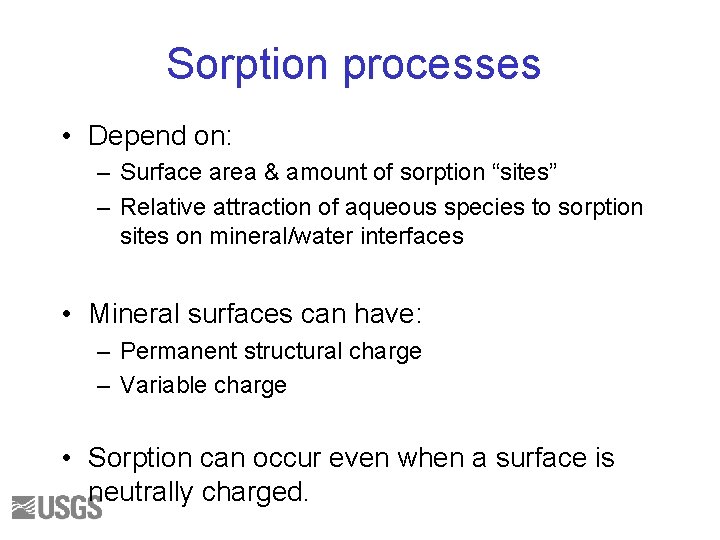
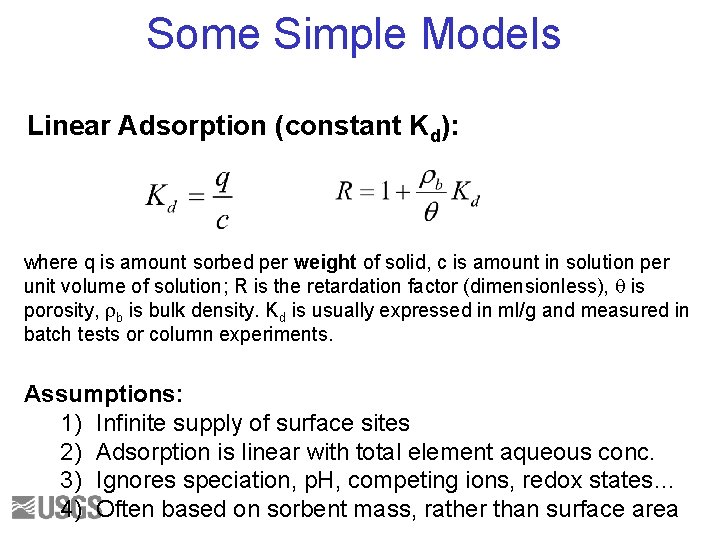
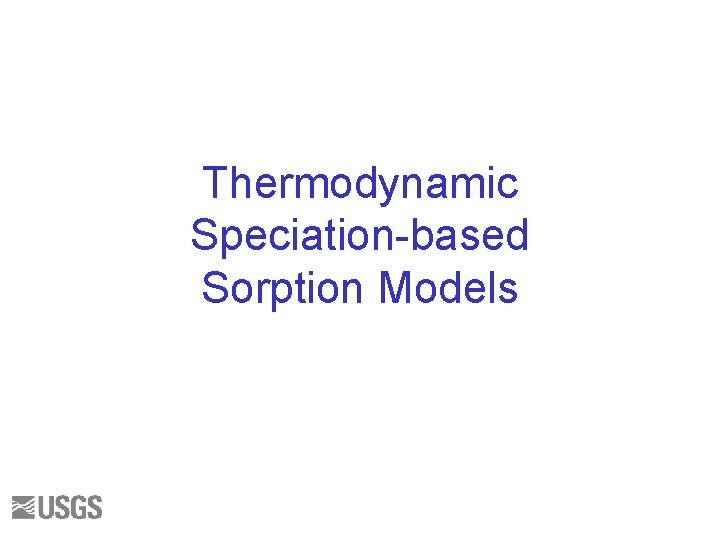
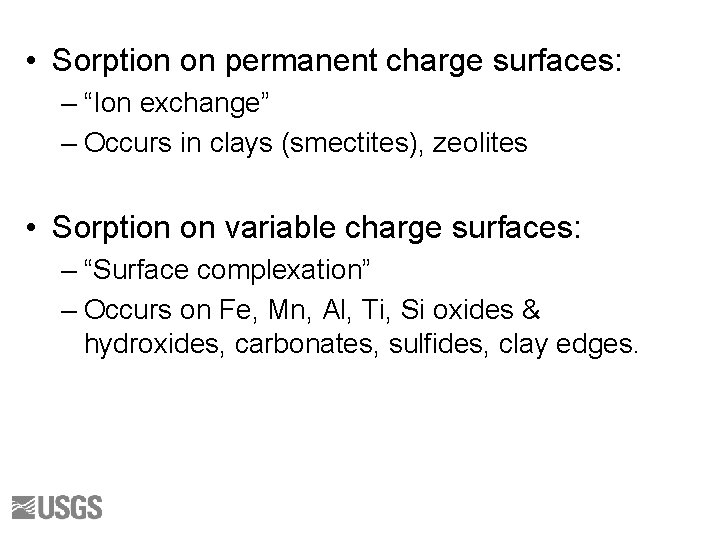
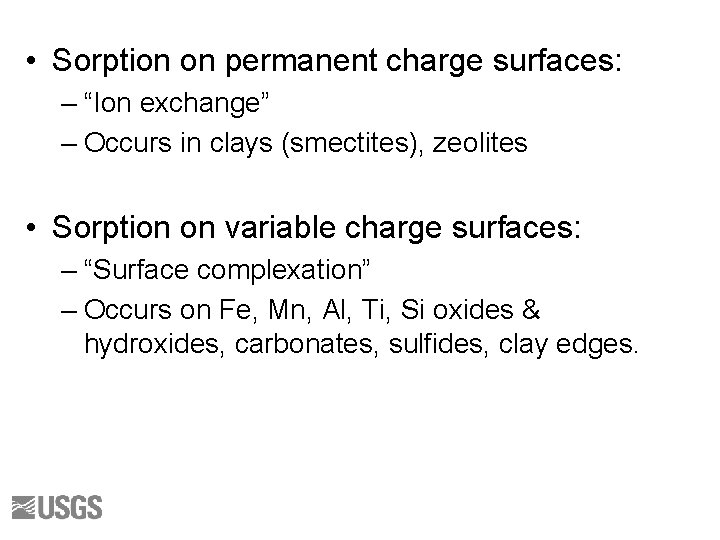
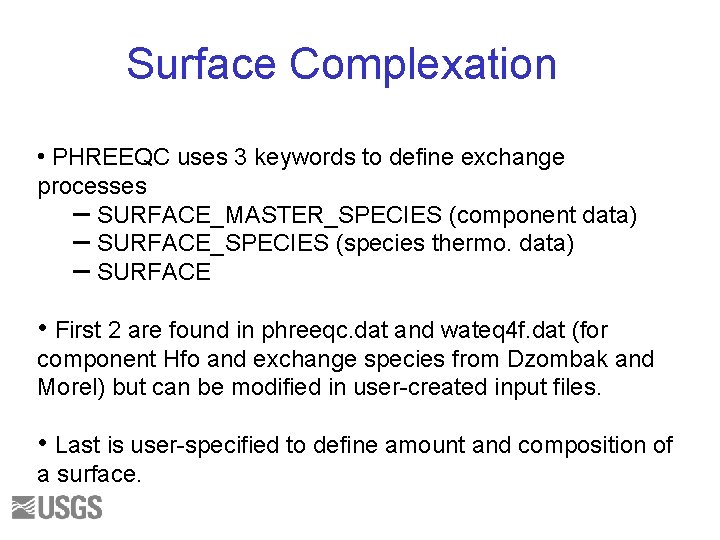
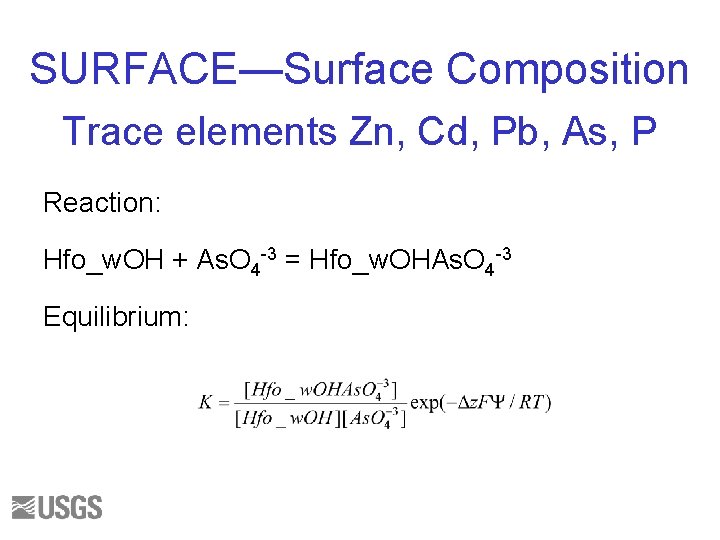
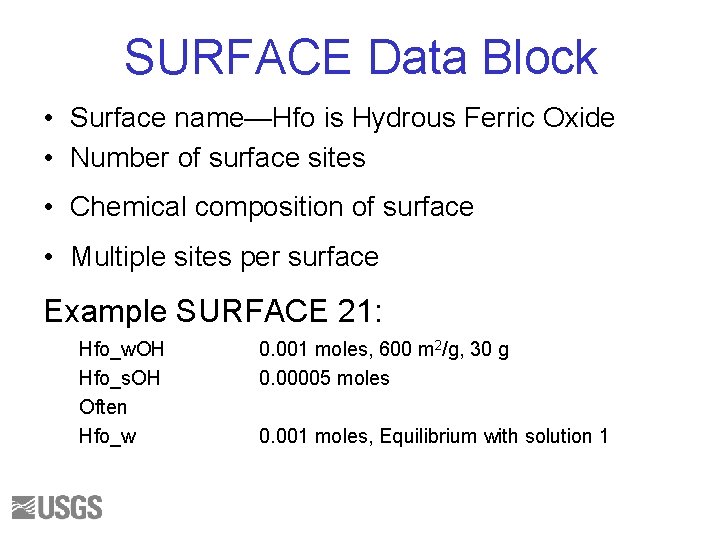
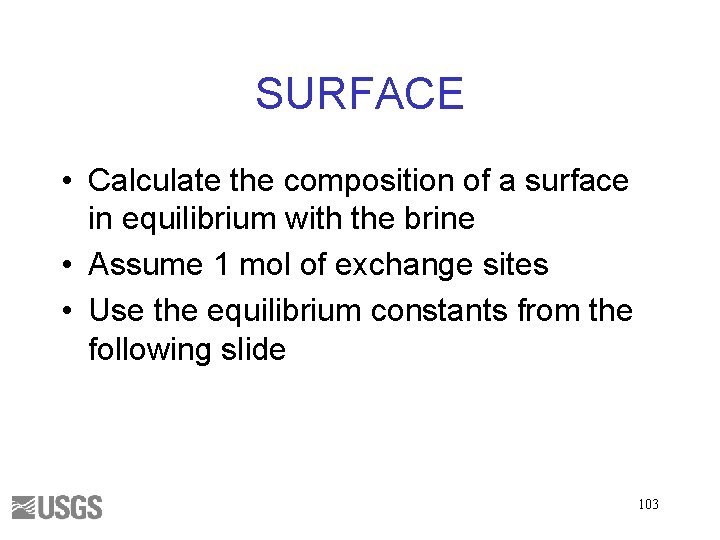
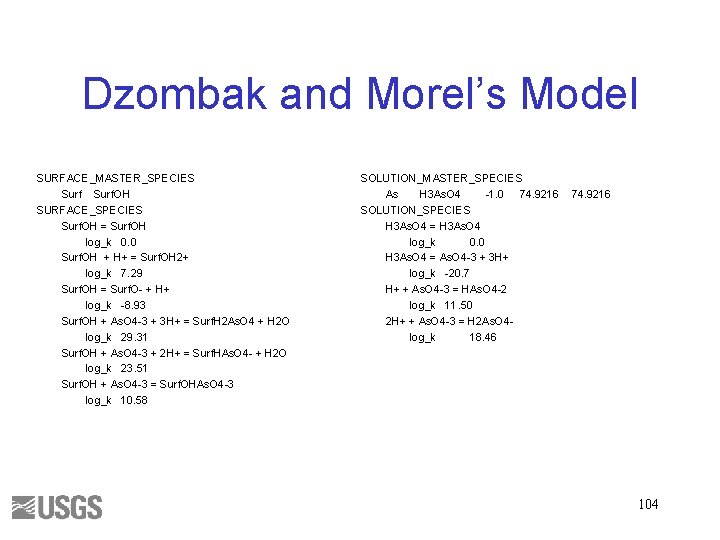
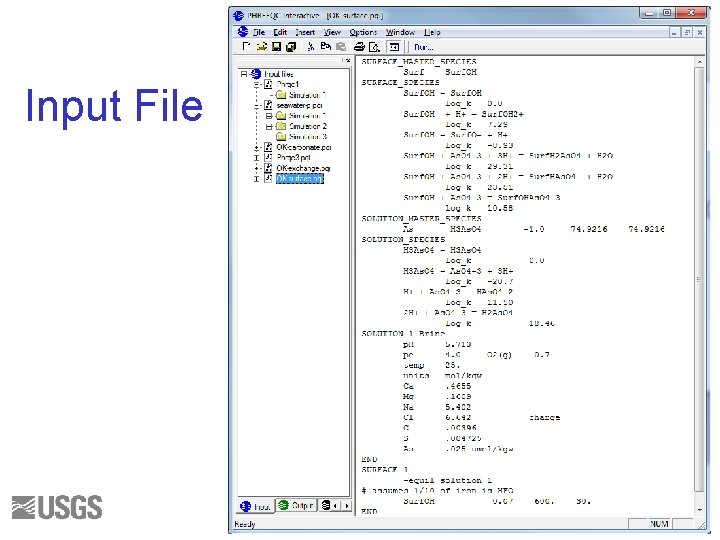
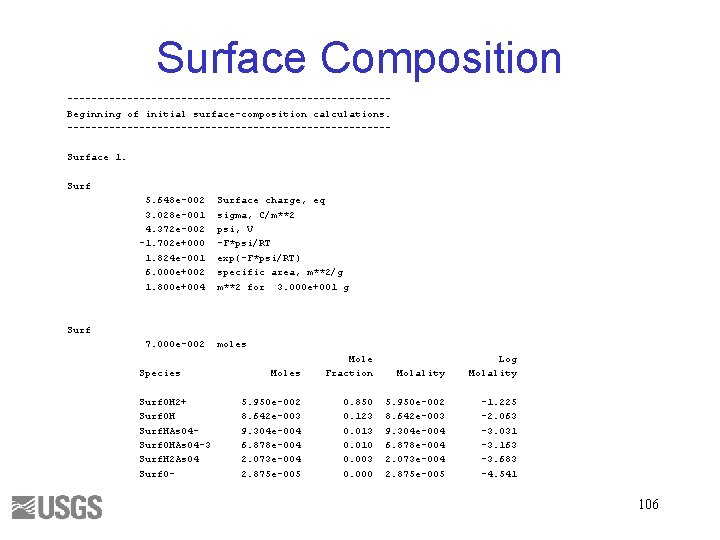
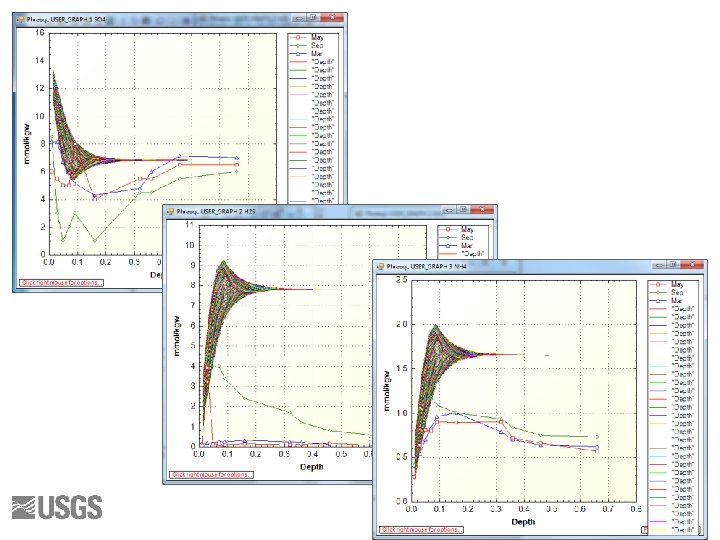
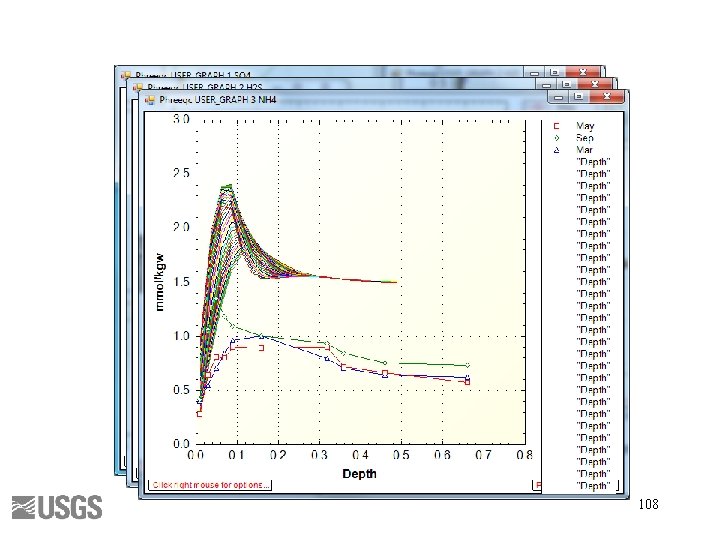
- Slides: 108
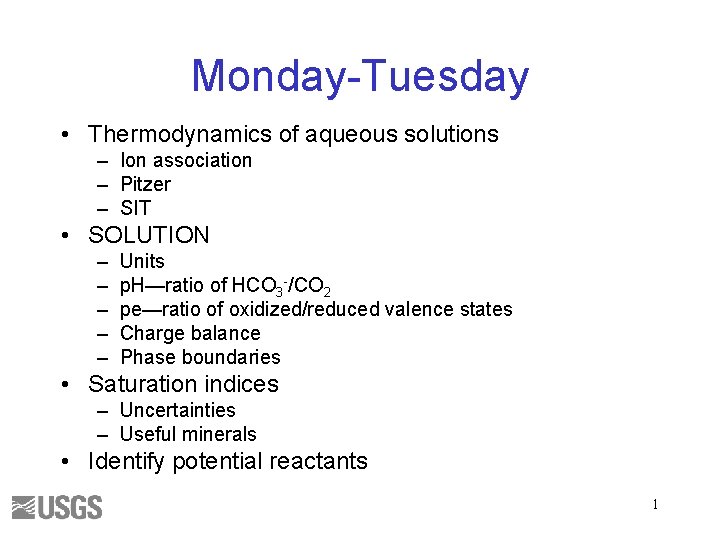
Monday-Tuesday • Thermodynamics of aqueous solutions – Ion association – Pitzer – SIT • SOLUTION – – – Units p. H—ratio of HCO 3 -/CO 2 pe—ratio of oxidized/reduced valence states Charge balance Phase boundaries • Saturation indices – Uncertainties – Useful minerals • Identify potential reactants 1
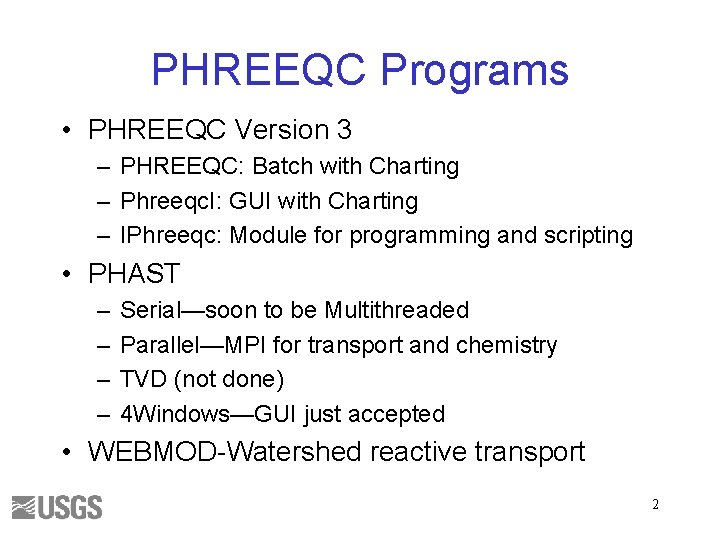
PHREEQC Programs • PHREEQC Version 3 – PHREEQC: Batch with Charting – Phreeqc. I: GUI with Charting – IPhreeqc: Module for programming and scripting • PHAST – – Serial—soon to be Multithreaded Parallel—MPI for transport and chemistry TVD (not done) 4 Windows—GUI just accepted • WEBMOD-Watershed reactive transport 2
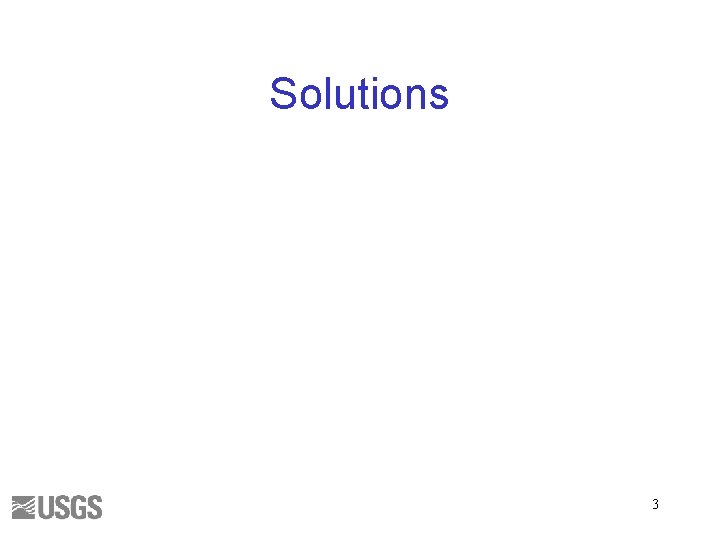
Solutions 3
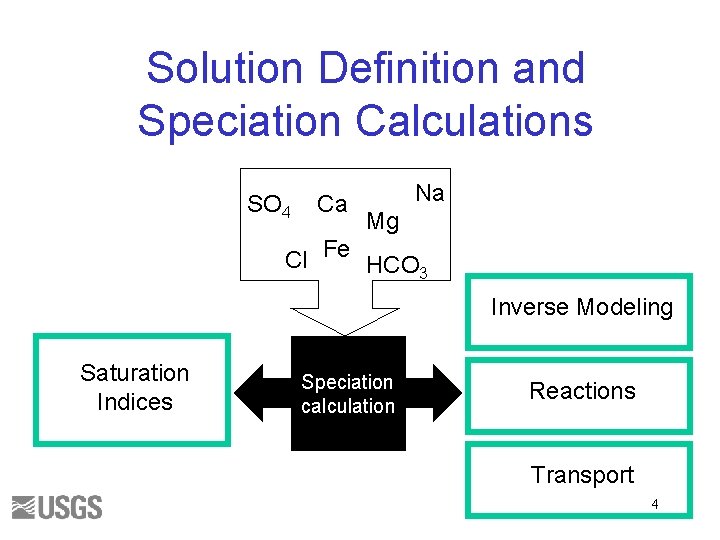
Solution Definition and Speciation Calculations SO 4 Ca Na Mg Cl Fe HCO 3 Inverse Modeling Saturation Indices Speciation calculation Reactions Transport 4
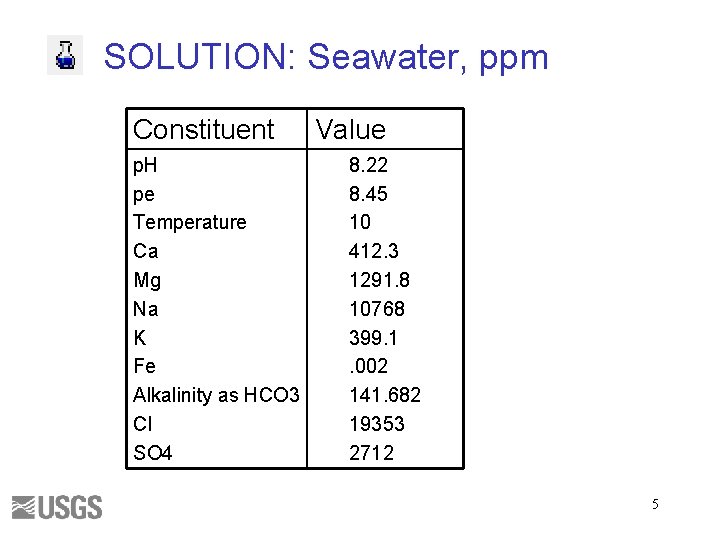
SOLUTION: Seawater, ppm Constituent p. H pe Temperature Ca Mg Na K Fe Alkalinity as HCO 3 Cl SO 4 Value 8. 22 8. 45 10 412. 3 1291. 8 10768 399. 1. 002 141. 682 19353 2712 5
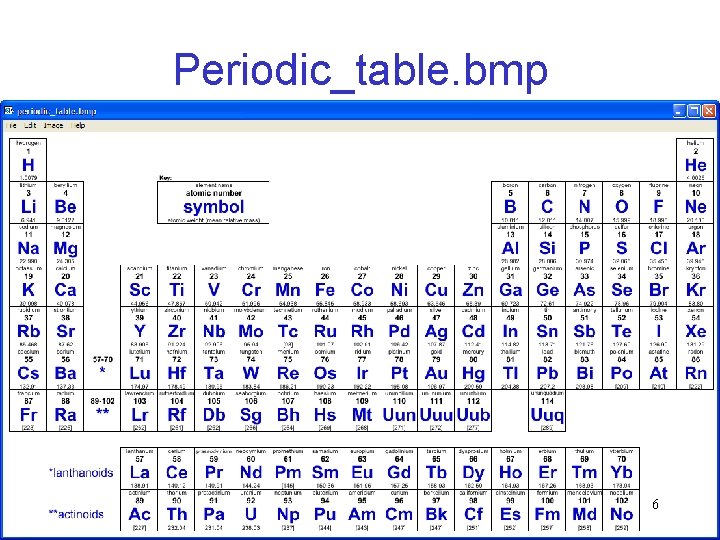
Periodic_table. bmp 6
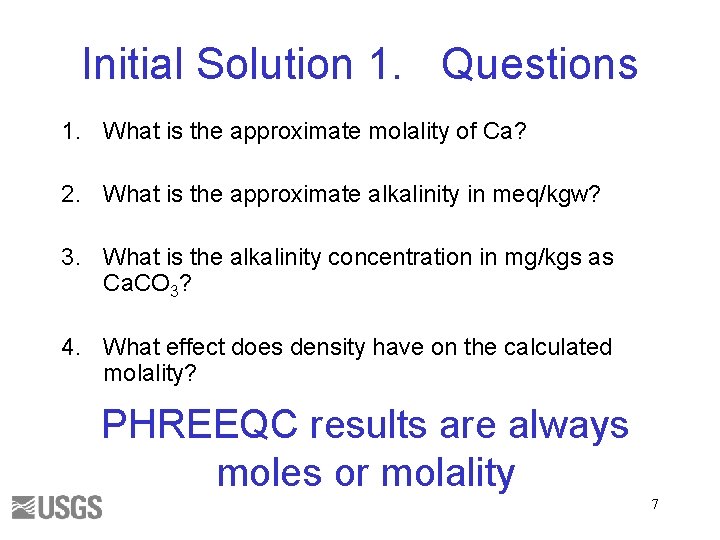
Initial Solution 1. Questions 1. What is the approximate molality of Ca? 2. What is the approximate alkalinity in meq/kgw? 3. What is the alkalinity concentration in mg/kgs as Ca. CO 3? 4. What effect does density have on the calculated molality? PHREEQC results are always moles or molality 7
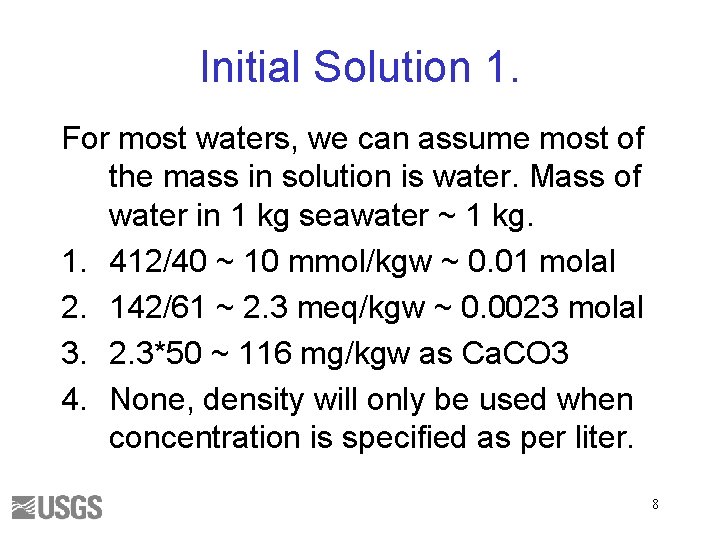
Initial Solution 1. For most waters, we can assume most of the mass in solution is water. Mass of water in 1 kg seawater ~ 1 kg. 1. 412/40 ~ 10 mmol/kgw ~ 0. 01 molal 2. 142/61 ~ 2. 3 meq/kgw ~ 0. 0023 molal 3. 2. 3*50 ~ 116 mg/kgw as Ca. CO 3 4. None, density will only be used when concentration is specified as per liter. 8
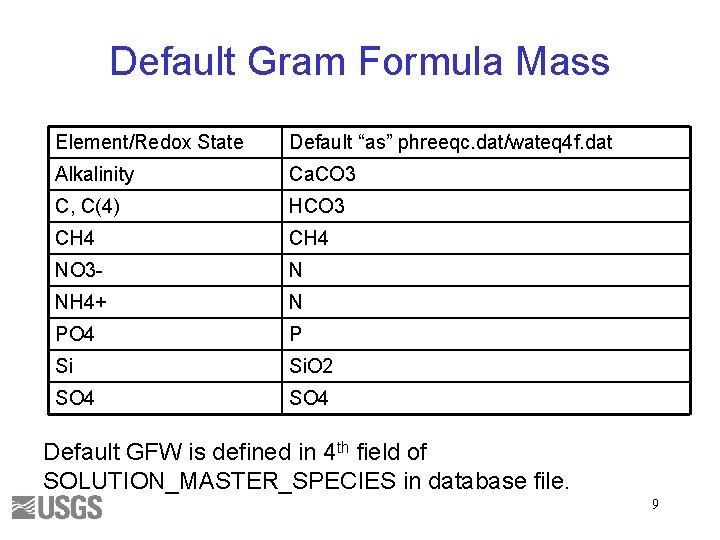
Default Gram Formula Mass Element/Redox State Default “as” phreeqc. dat/wateq 4 f. dat Alkalinity Ca. CO 3 C, C(4) HCO 3 CH 4 NO 3 - N NH 4+ N PO 4 P Si Si. O 2 SO 4 Default GFW is defined in 4 th field of SOLUTION_MASTER_SPECIES in database file. 9
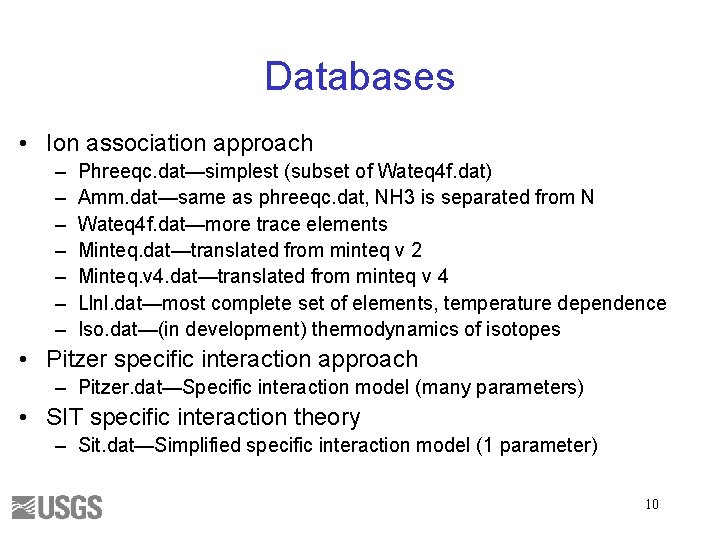
Databases • Ion association approach – – – – Phreeqc. dat—simplest (subset of Wateq 4 f. dat) Amm. dat—same as phreeqc. dat, NH 3 is separated from N Wateq 4 f. dat—more trace elements Minteq. dat—translated from minteq v 2 Minteq. v 4. dat—translated from minteq v 4 Llnl. dat—most complete set of elements, temperature dependence Iso. dat—(in development) thermodynamics of isotopes • Pitzer specific interaction approach – Pitzer. dat—Specific interaction model (many parameters) • SIT specific interaction theory – Sit. dat—Simplified specific interaction model (1 parameter) 10
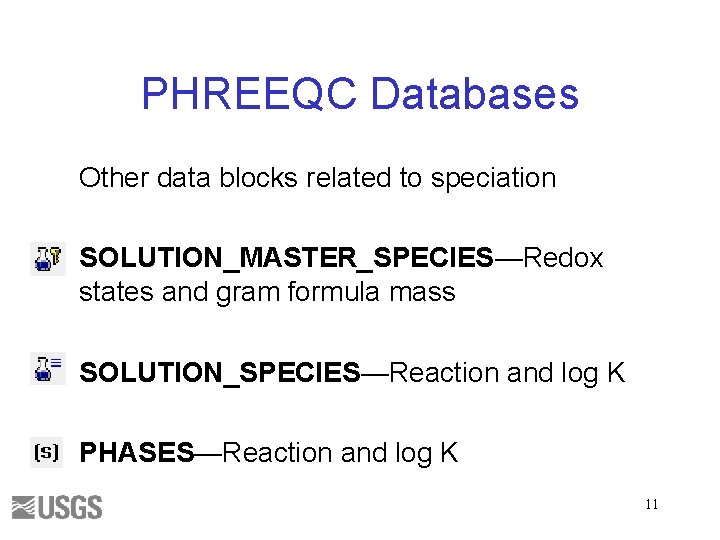
PHREEQC Databases Other data blocks related to speciation SOLUTION_MASTER_SPECIES—Redox states and gram formula mass SOLUTION_SPECIES—Reaction and log K PHASES—Reaction and log K 11
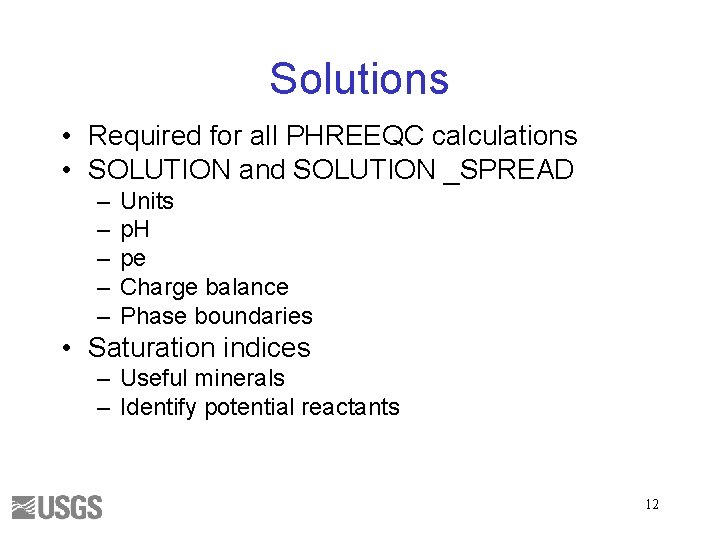
Solutions • Required for all PHREEQC calculations • SOLUTION and SOLUTION _SPREAD – – – Units p. H pe Charge balance Phase boundaries • Saturation indices – Useful minerals – Identify potential reactants 12
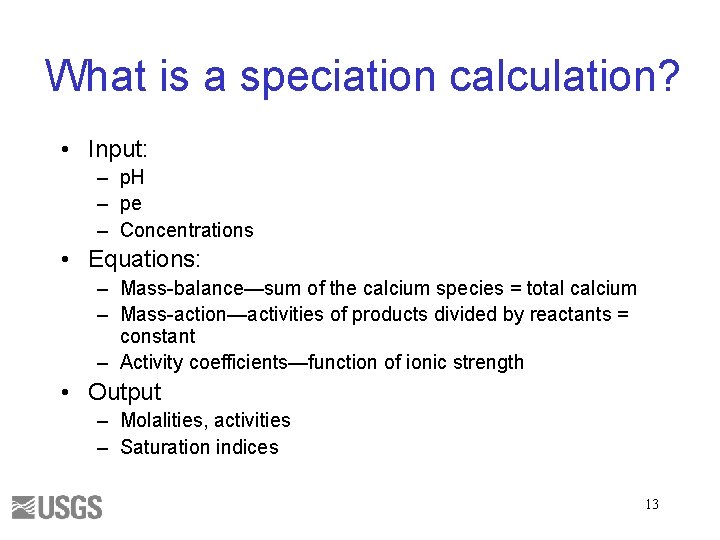
What is a speciation calculation? • Input: – p. H – pe – Concentrations • Equations: – Mass-balance—sum of the calcium species = total calcium – Mass-action—activities of products divided by reactants = constant – Activity coefficients—function of ionic strength • Output – Molalities, activities – Saturation indices 13
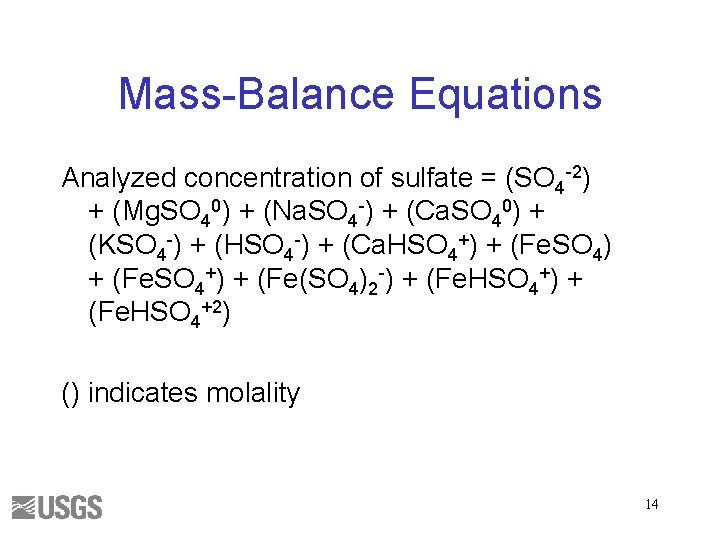
Mass-Balance Equations Analyzed concentration of sulfate = (SO 4 -2) + (Mg. SO 40) + (Na. SO 4 -) + (Ca. SO 40) + (KSO 4 -) + (HSO 4 -) + (Ca. HSO 4+) + (Fe(SO 4)2 -) + (Fe. HSO 4+2) () indicates molality 14
![MassAction Equations Ca2 SO 4 2 Ca SO 40 indicates activity Mass-Action Equations Ca+2 + SO 4 -2 = Ca. SO 40 [] indicates activity](https://slidetodoc.com/presentation_image/200b8de3ad78ea91e8cd99b28236b61f/image-15.jpg)
Mass-Action Equations Ca+2 + SO 4 -2 = Ca. SO 40 [] indicates activity 15
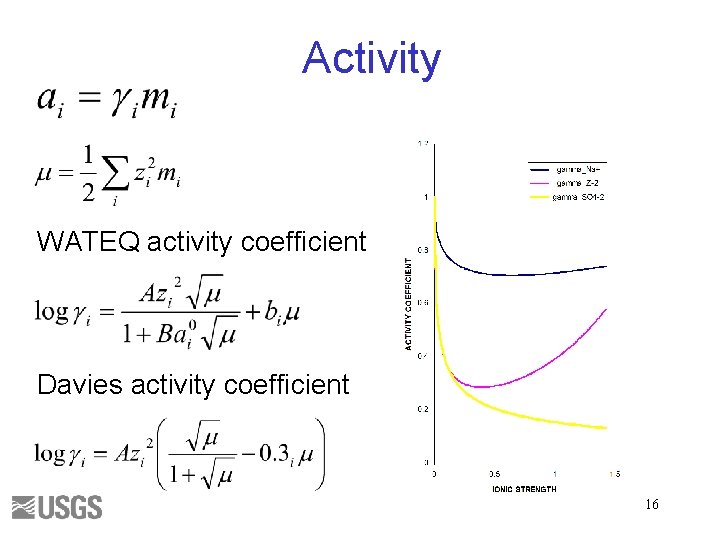
Activity WATEQ activity coefficient Davies activity coefficient 16
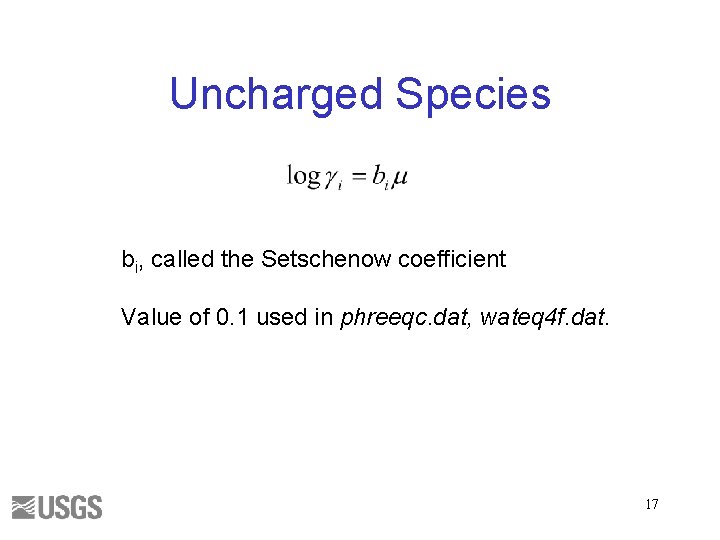
Uncharged Species bi, called the Setschenow coefficient Value of 0. 1 used in phreeqc. dat, wateq 4 f. dat. 17
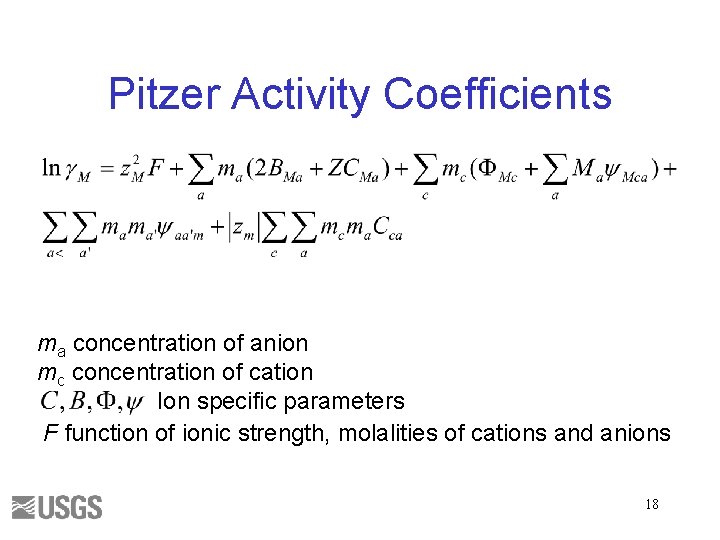
Pitzer Activity Coefficients ma concentration of anion mc concentration of cation Ion specific parameters F function of ionic strength, molalities of cations and anions 18
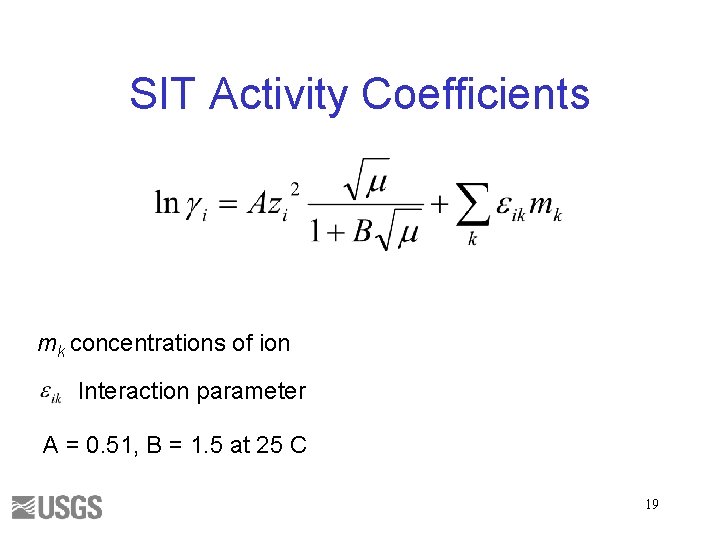
SIT Activity Coefficients mk concentrations of ion Interaction parameter A = 0. 51, B = 1. 5 at 25 C 19
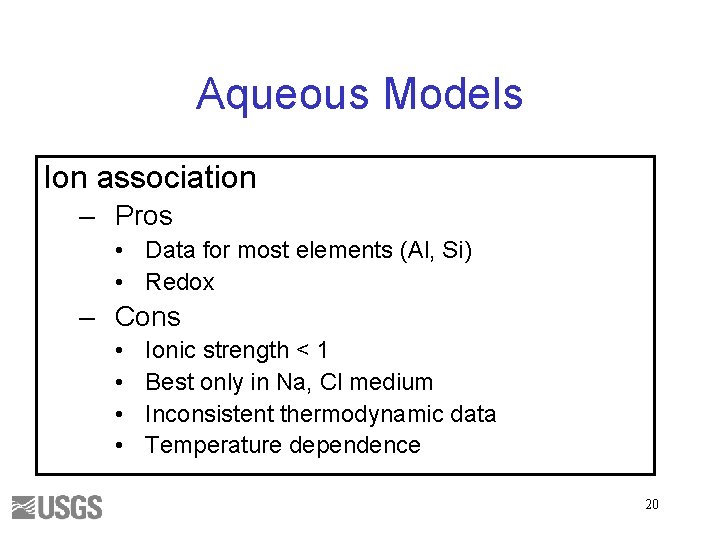
Aqueous Models Ion association – Pros • Data for most elements (Al, Si) • Redox – Cons • • Ionic strength < 1 Best only in Na, Cl medium Inconsistent thermodynamic data Temperature dependence 20
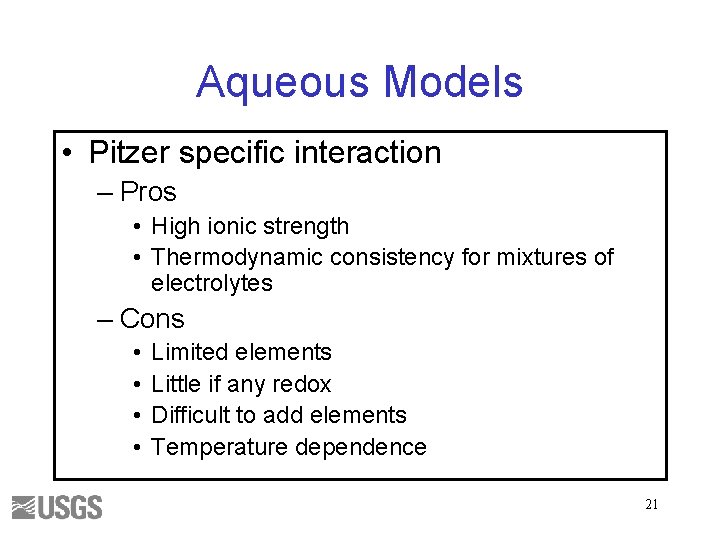
Aqueous Models • Pitzer specific interaction – Pros • High ionic strength • Thermodynamic consistency for mixtures of electrolytes – Cons • • Limited elements Little if any redox Difficult to add elements Temperature dependence 21
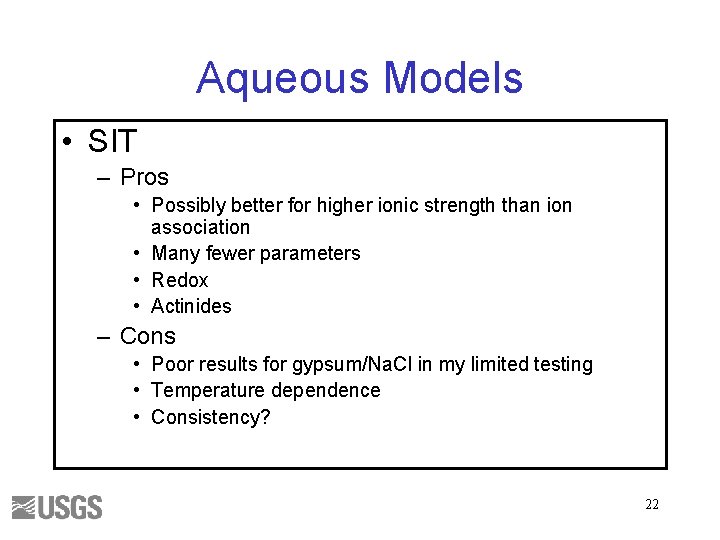
Aqueous Models • SIT – Pros • Possibly better for higher ionic strength than ion association • Many fewer parameters • Redox • Actinides – Cons • Poor results for gypsum/Na. Cl in my limited testing • Temperature dependence • Consistency? 22
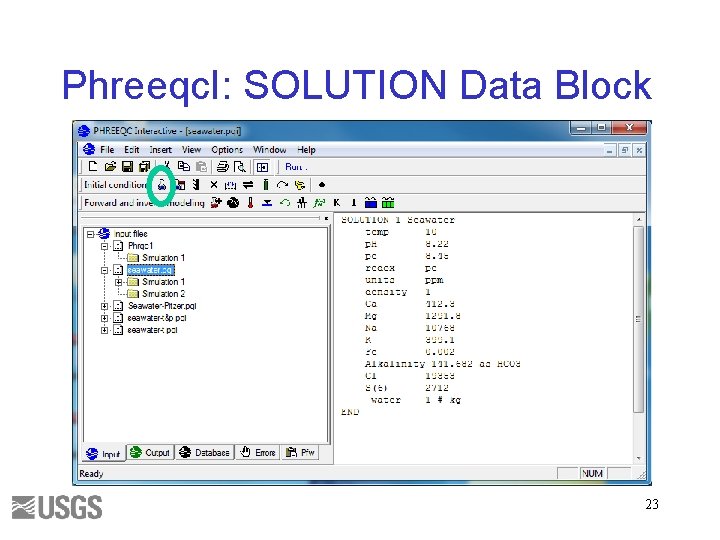
Phreeqc. I: SOLUTION Data Block 23
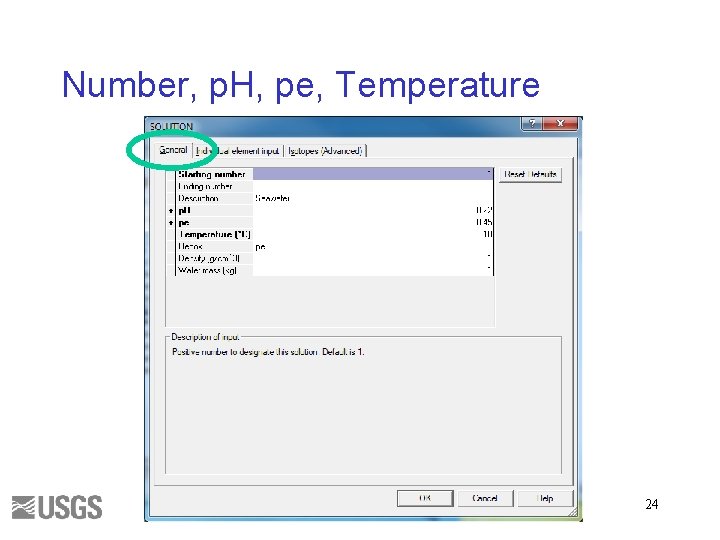
Number, p. H, pe, Temperature 24
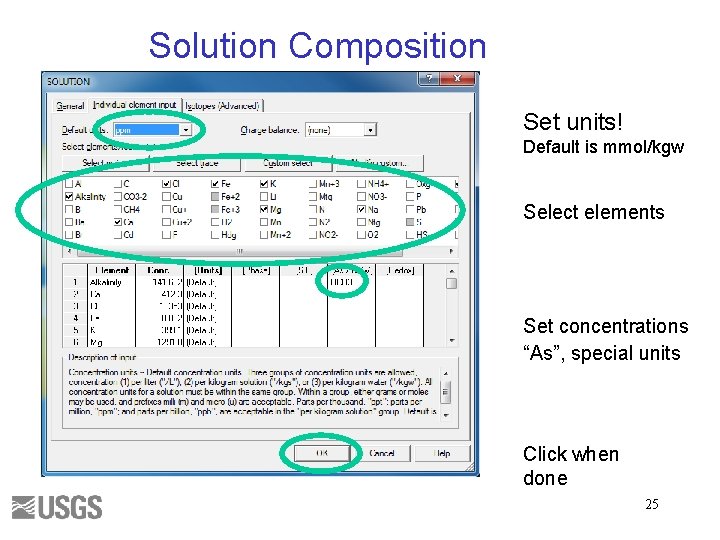
Solution Composition Set units! Default is mmol/kgw Select elements Set concentrations “As”, special units Click when done 25
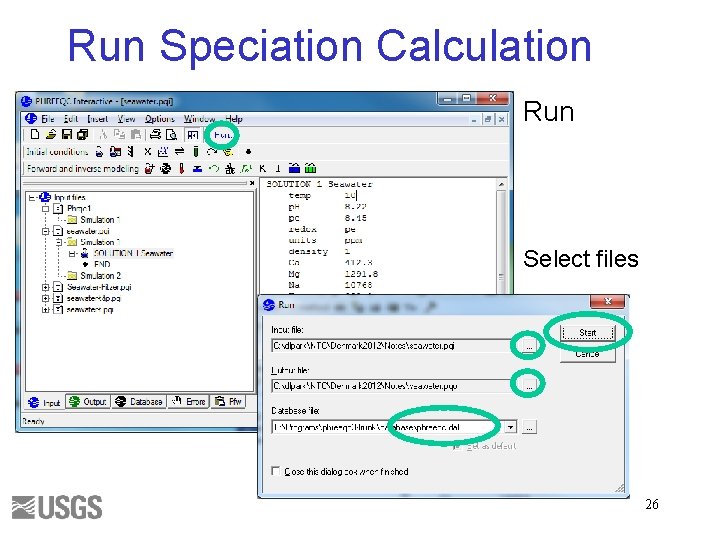
Run Speciation Calculation Run Select files 26
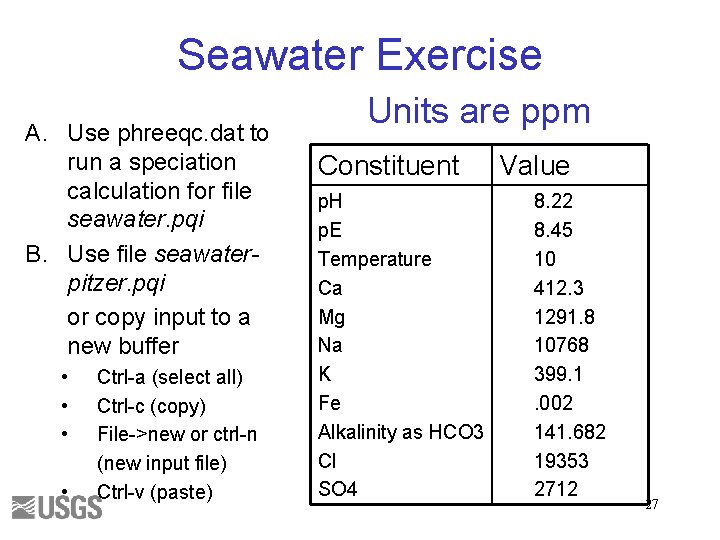
Seawater Exercise A. Use phreeqc. dat to run a speciation calculation for file seawater. pqi B. Use file seawaterpitzer. pqi or copy input to a new buffer • • Ctrl-a (select all) Ctrl-c (copy) File->new or ctrl-n (new input file) Ctrl-v (paste) Units are ppm Constituent p. H p. E Temperature Ca Mg Na K Fe Alkalinity as HCO 3 Cl SO 4 Value 8. 22 8. 45 10 412. 3 1291. 8 10768 399. 1. 002 141. 682 19353 2712 27
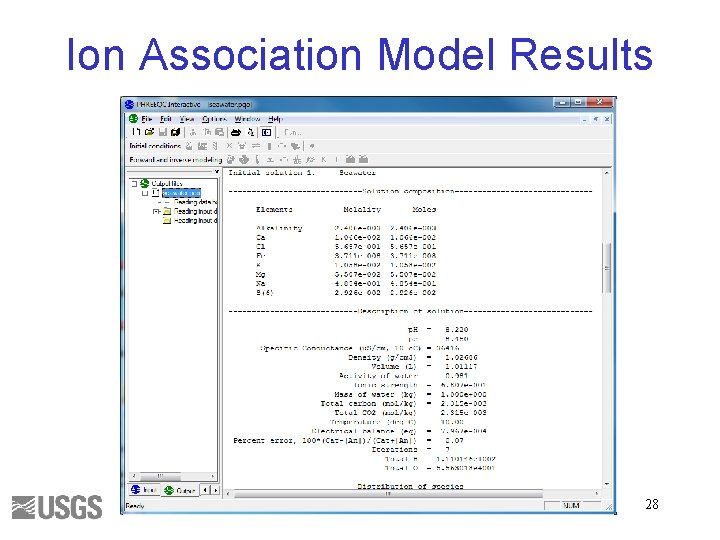
Ion Association Model Results 28
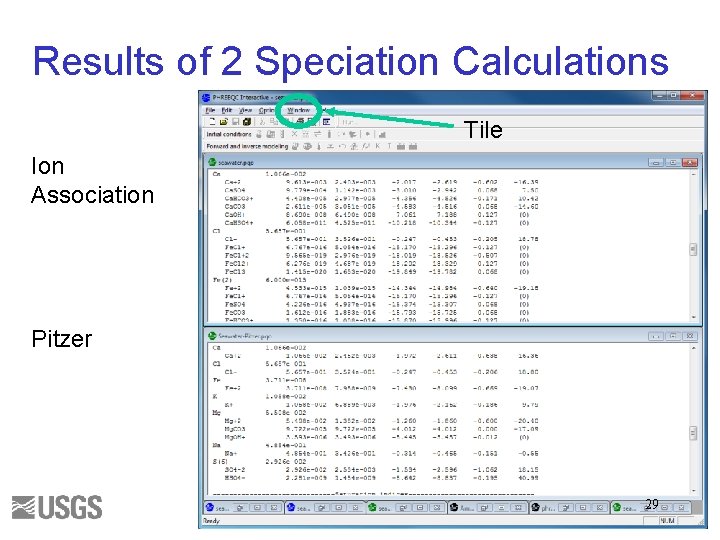
Results of 2 Speciation Calculations Tile Ion Association Pitzer 29
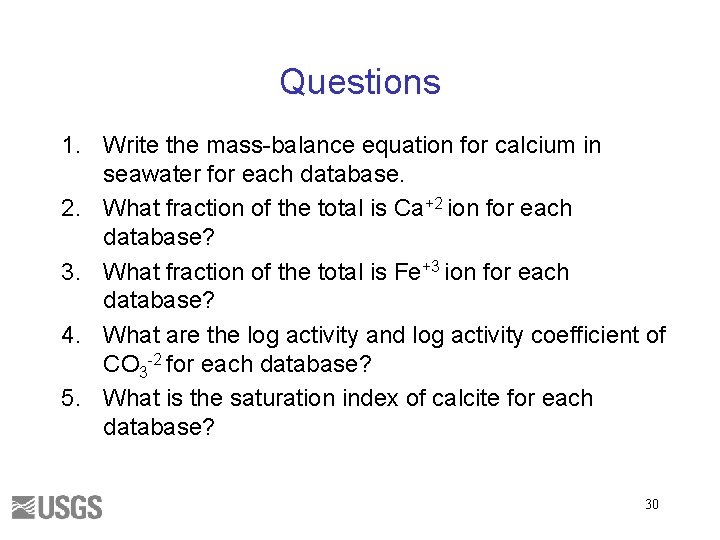
Questions 1. Write the mass-balance equation for calcium in seawater for each database. 2. What fraction of the total is Ca+2 ion for each database? 3. What fraction of the total is Fe+3 ion for each database? 4. What are the log activity and log activity coefficient of CO 3 -2 for each database? 5. What is the saturation index of calcite for each database? 30
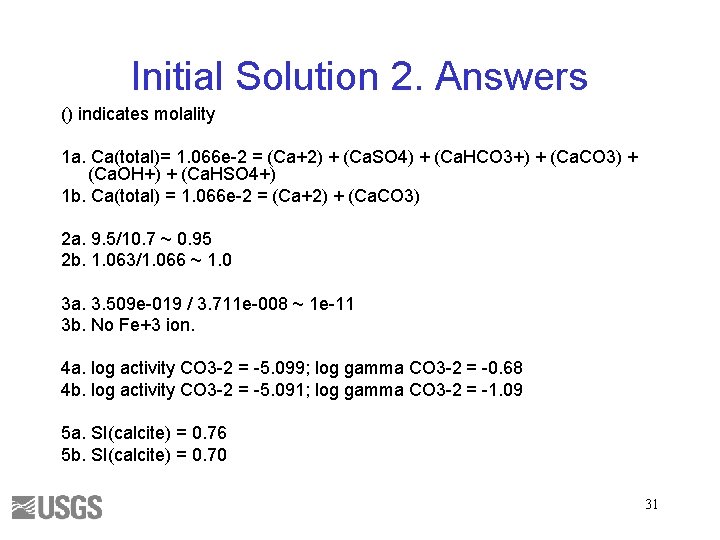
Initial Solution 2. Answers () indicates molality 1 a. Ca(total)= 1. 066 e-2 = (Ca+2) + (Ca. SO 4) + (Ca. HCO 3+) + (Ca. CO 3) + (Ca. OH+) + (Ca. HSO 4+) 1 b. Ca(total) = 1. 066 e-2 = (Ca+2) + (Ca. CO 3) 2 a. 9. 5/10. 7 ~ 0. 95 2 b. 1. 063/1. 066 ~ 1. 0 3 a. 3. 509 e-019 / 3. 711 e-008 ~ 1 e-11 3 b. No Fe+3 ion. 4 a. log activity CO 3 -2 = -5. 099; log gamma CO 3 -2 = -0. 68 4 b. log activity CO 3 -2 = -5. 091; log gamma CO 3 -2 = -1. 09 5 a. SI(calcite) = 0. 76 5 b. SI(calcite) = 0. 70 31
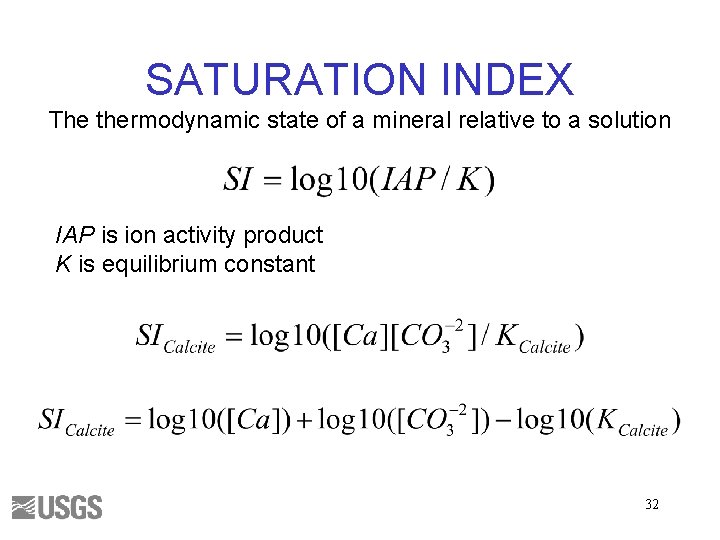
SATURATION INDEX The thermodynamic state of a mineral relative to a solution IAP is ion activity product K is equilibrium constant 32
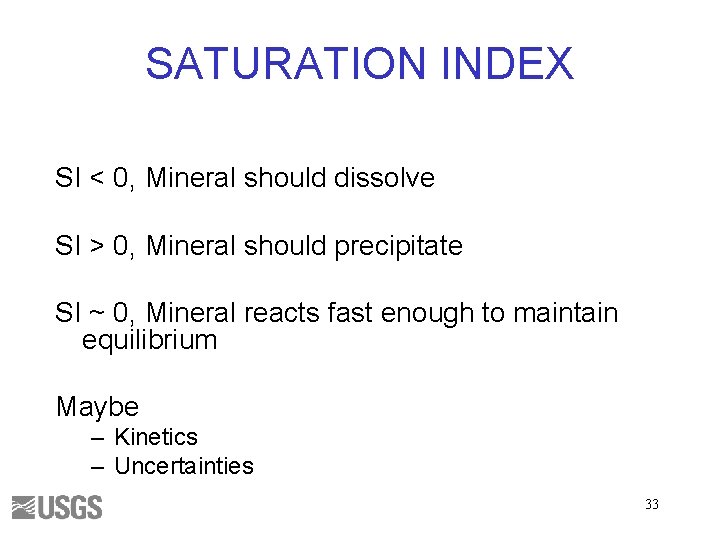
SATURATION INDEX SI < 0, Mineral should dissolve SI > 0, Mineral should precipitate SI ~ 0, Mineral reacts fast enough to maintain equilibrium Maybe – Kinetics – Uncertainties 33
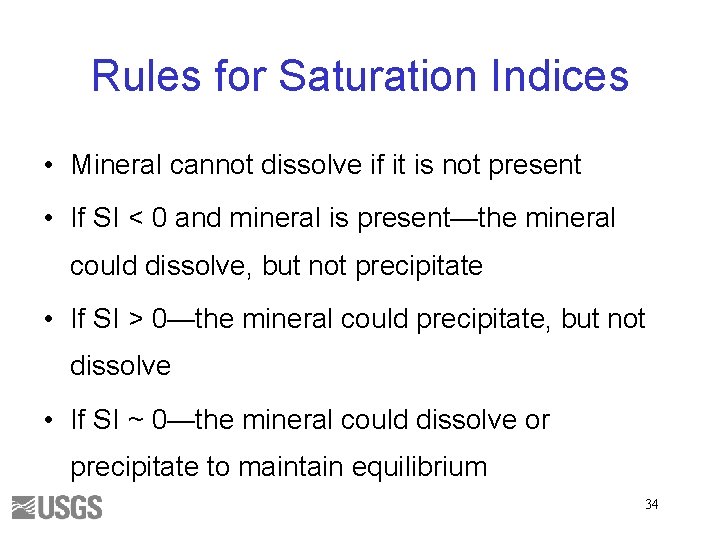
Rules for Saturation Indices • Mineral cannot dissolve if it is not present • If SI < 0 and mineral is present—the mineral could dissolve, but not precipitate • If SI > 0—the mineral could precipitate, but not dissolve • If SI ~ 0—the mineral could dissolve or precipitate to maintain equilibrium 34
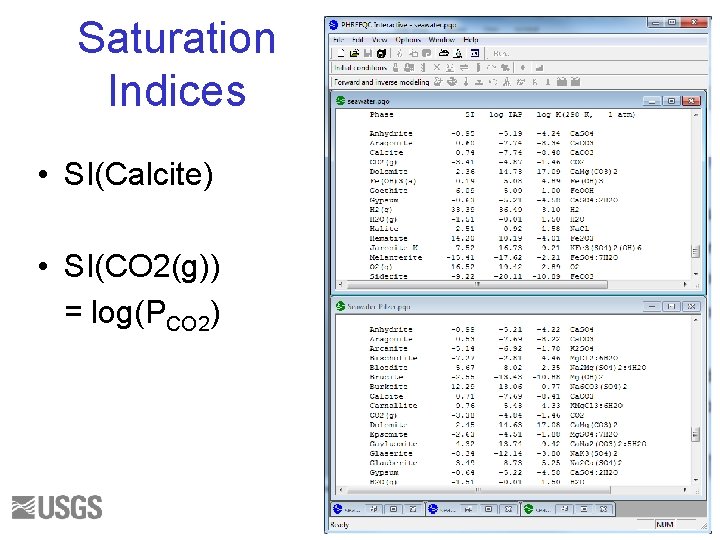
Saturation Indices • SI(Calcite) • SI(CO 2(g)) = log(PCO 2) 35
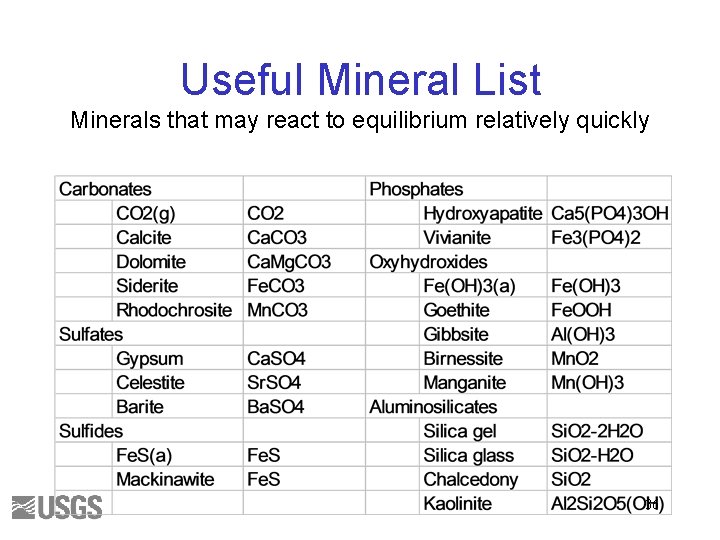
Useful Mineral List Minerals that may react to equilibrium relatively quickly 36
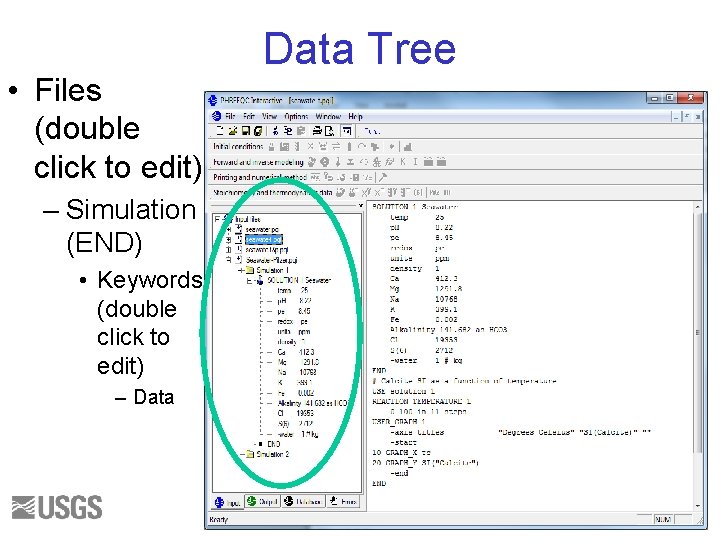
• Files (double click to edit) Data Tree – Simulation (END) • Keywords (double click to edit) – Data 37
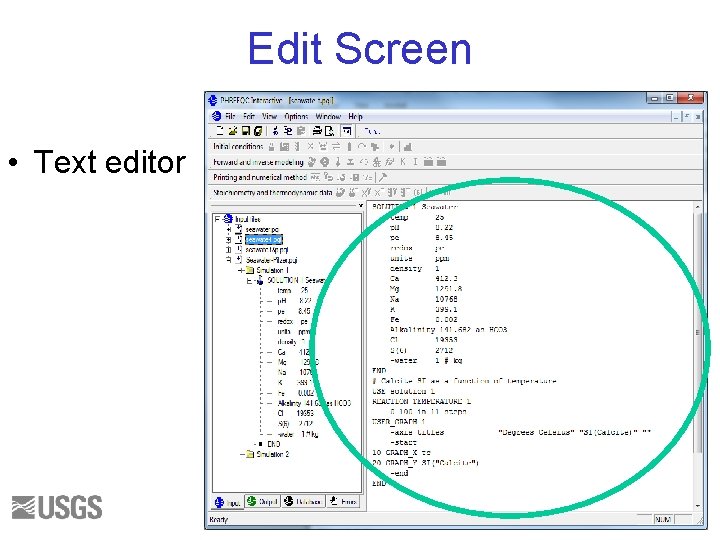
Edit Screen • Text editor 38
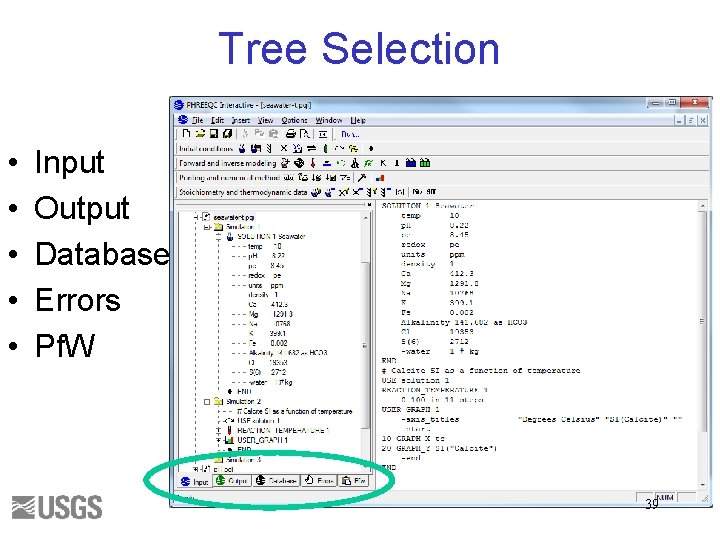
Tree Selection • • • Input Output Database Errors Pf. W 39
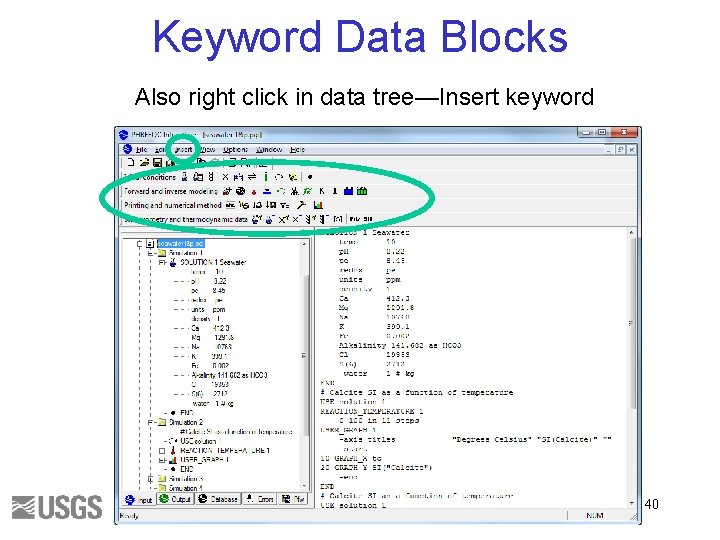
Keyword Data Blocks Also right click in data tree—Insert keyword 40
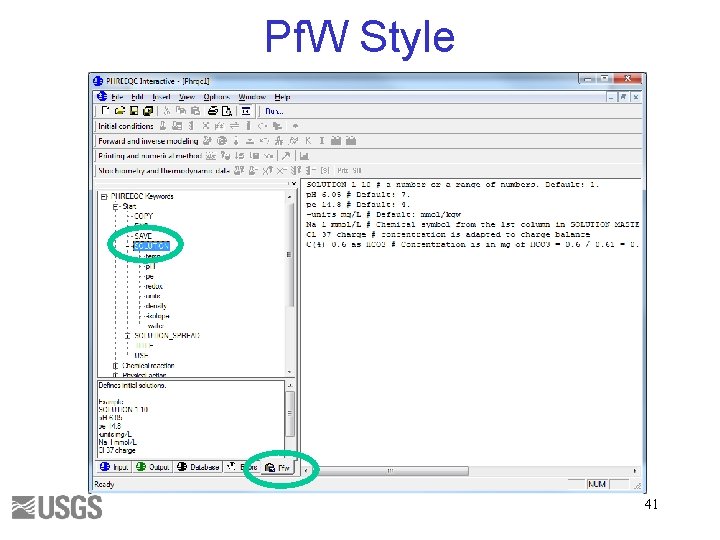
Pf. W Style 41
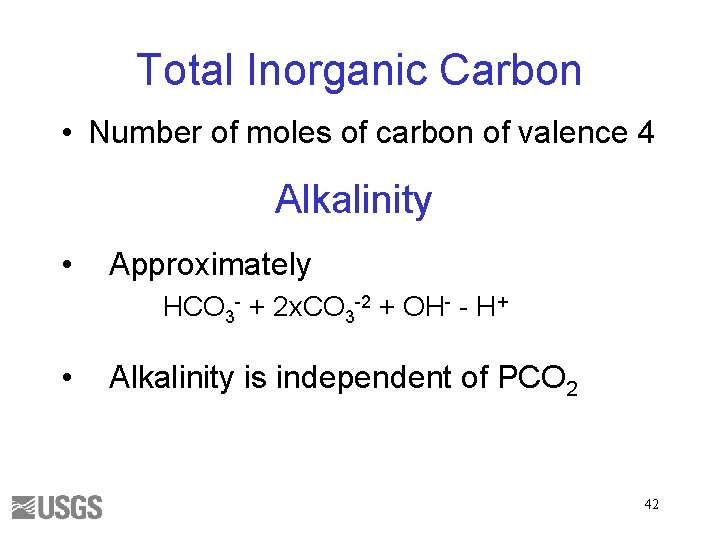
Total Inorganic Carbon • Number of moles of carbon of valence 4 Alkalinity • Approximately HCO 3 - + 2 x. CO 3 -2 + OH- - H+ • Alkalinity is independent of PCO 2 42
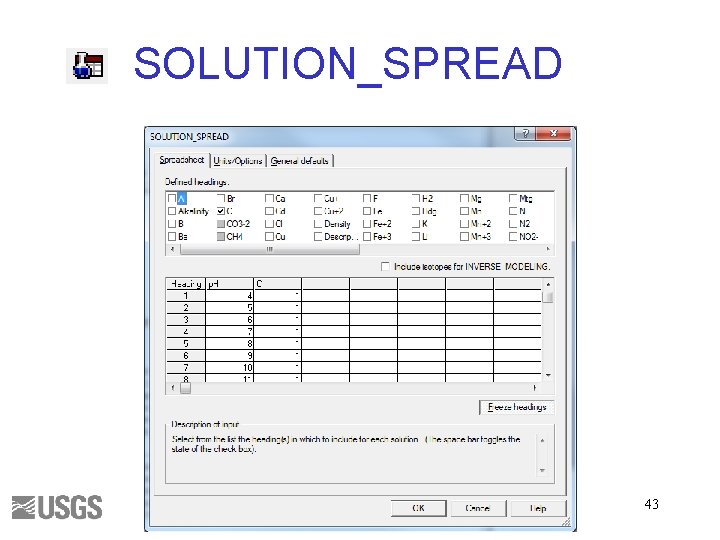
SOLUTION_SPREAD 43
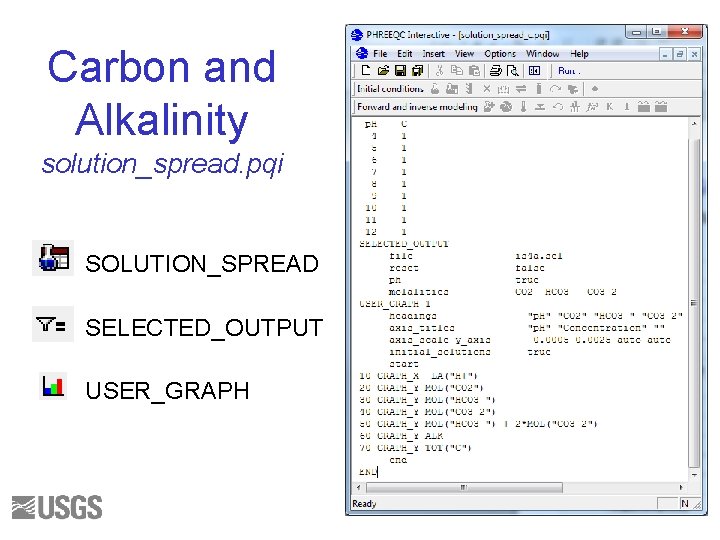
Carbon and Alkalinity solution_spread. pqi SOLUTION_SPREAD SELECTED_OUTPUT USER_GRAPH 44
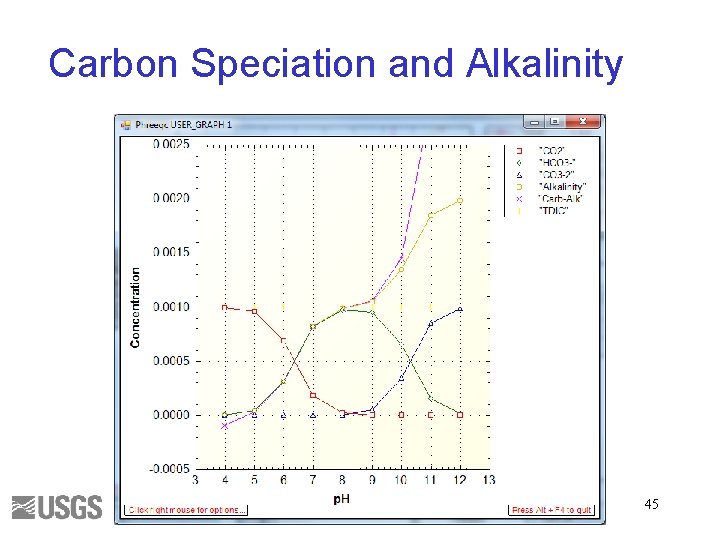
Carbon Speciation and Alkalinity 45
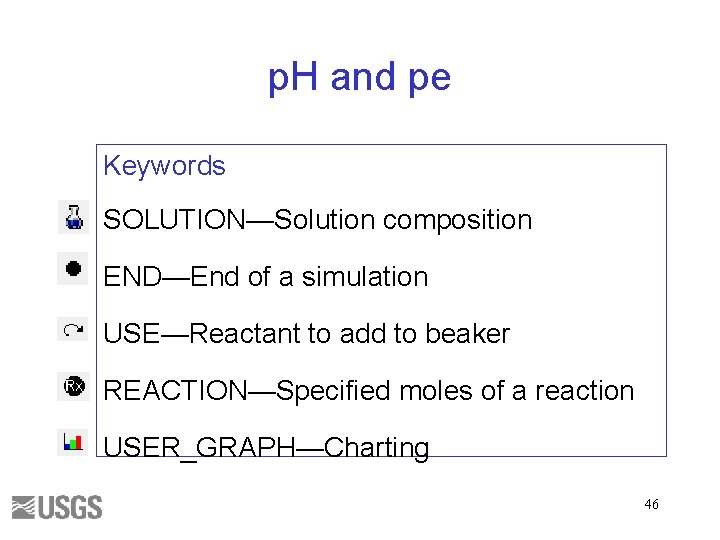
p. H and pe Keywords SOLUTION—Solution composition END—End of a simulation USE—Reactant to add to beaker REACTION—Specified moles of a reaction USER_GRAPH—Charting 46
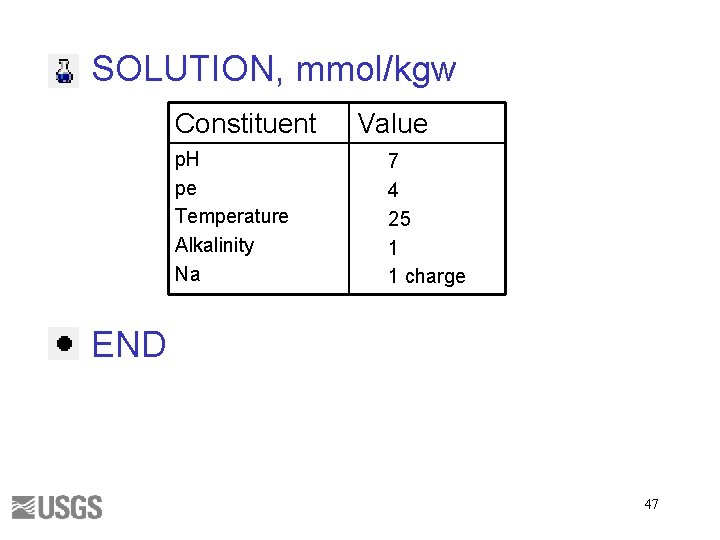
SOLUTION, mmol/kgw Constituent p. H pe Temperature Alkalinity Na Value 7 4 25 1 1 charge END 47
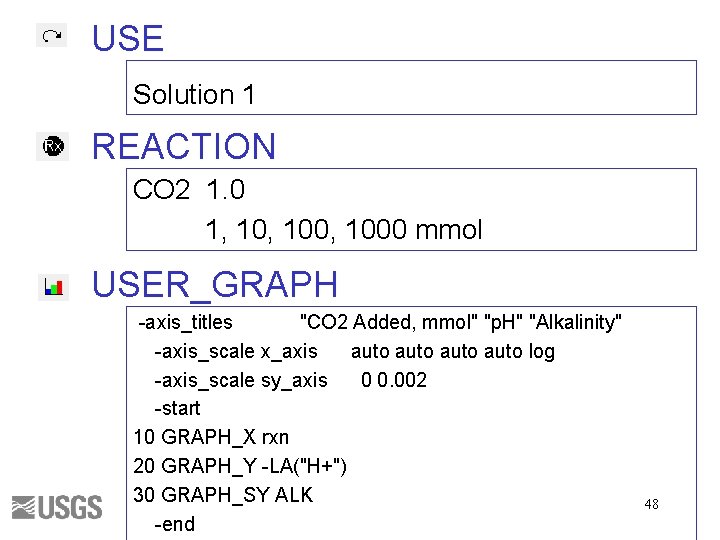
USE Solution 1 REACTION CO 2 1. 0 1, 100, 1000 mmol USER_GRAPH -axis_titles "CO 2 Added, mmol" "p. H" "Alkalinity" -axis_scale x_axis auto log -axis_scale sy_axis 0 0. 002 -start 10 GRAPH_X rxn 20 GRAPH_Y -LA("H+") 30 GRAPH_SY ALK -end 48
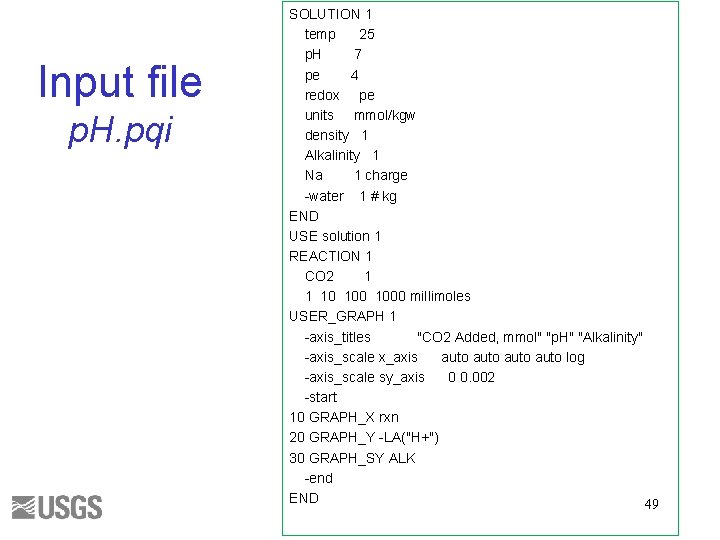
Input file p. H. pqi SOLUTION 1 temp 25 p. H 7 pe 4 redox pe units mmol/kgw density 1 Alkalinity 1 Na 1 charge -water 1 # kg END USE solution 1 REACTION 1 CO 2 1 1 10 1000 millimoles USER_GRAPH 1 -axis_titles "CO 2 Added, mmol" "p. H" "Alkalinity" -axis_scale x_axis auto log -axis_scale sy_axis 0 0. 002 -start 10 GRAPH_X rxn 20 GRAPH_Y -LA("H+") 30 GRAPH_SY ALK -end END 49
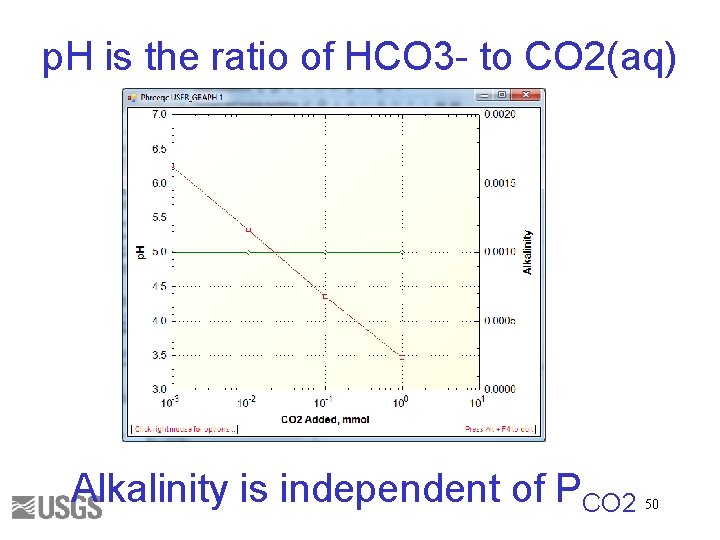
p. H is the ratio of HCO 3 - to CO 2(aq) Alkalinity is independent of PCO 2 50
![What is p H p H 6 3 logHCO 3 CO 2 What is p. H? p. H = 6. 3 + log[(HCO 3 -)/(CO 2)]](https://slidetodoc.com/presentation_image/200b8de3ad78ea91e8cd99b28236b61f/image-51.jpg)
What is p. H? p. H = 6. 3 + log[(HCO 3 -)/(CO 2)] p. H = 10. 3 + log[(CO 3 -2)/(HCO 3 -)] p. H = log. K + log[(PO 4 -3)/(HPO 4 -2)] Questions 1. How does the p. H change when CO 2 degasses during an alkalinity titration? 2. How does p. H change when plankton respire CO 2? 3. How does p. H change when calcite dissolves? 51
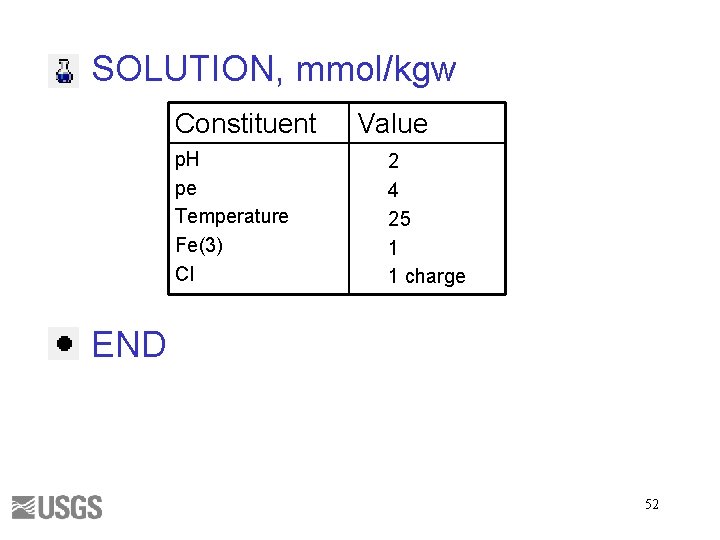
SOLUTION, mmol/kgw Constituent p. H pe Temperature Fe(3) Cl Value 2 4 25 1 1 charge END 52
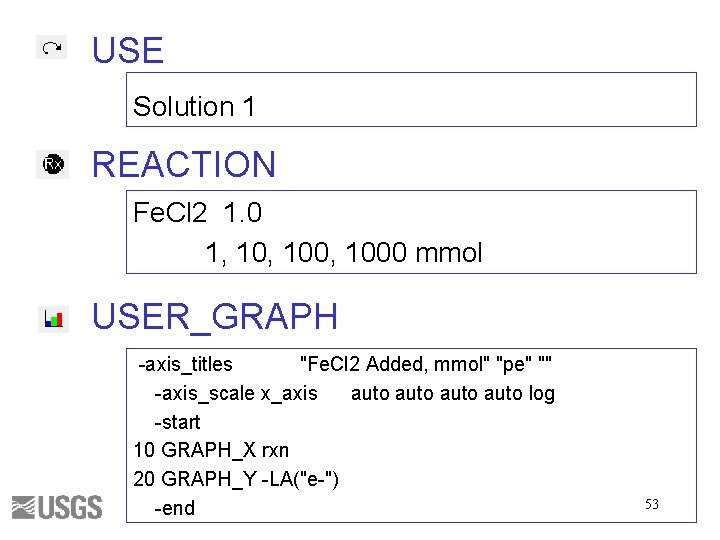
USE Solution 1 REACTION Fe. Cl 2 1. 0 1, 100, 1000 mmol USER_GRAPH -axis_titles "Fe. Cl 2 Added, mmol" "pe" "" -axis_scale x_axis auto log -start 10 GRAPH_X rxn 20 GRAPH_Y -LA("e-") -end 53
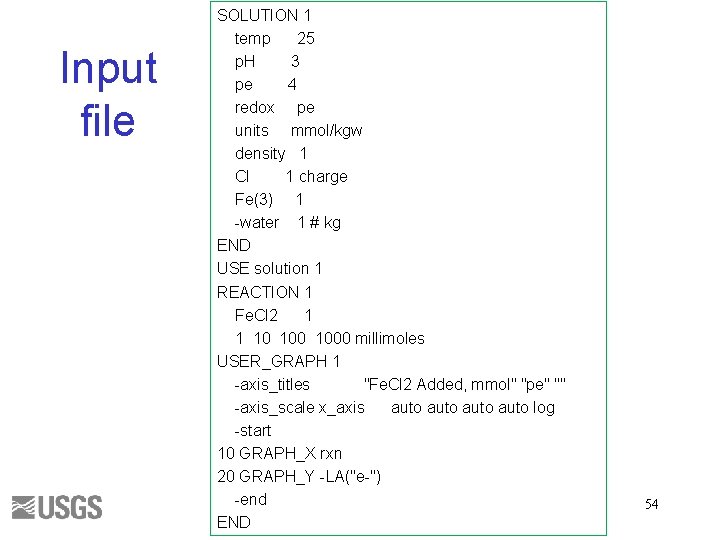
Input file SOLUTION 1 temp 25 p. H 3 pe 4 redox pe units mmol/kgw density 1 Cl 1 charge Fe(3) 1 -water 1 # kg END USE solution 1 REACTION 1 Fe. Cl 2 1 1 10 1000 millimoles USER_GRAPH 1 -axis_titles "Fe. Cl 2 Added, mmol" "pe" "" -axis_scale x_axis auto log -start 10 GRAPH_X rxn 20 GRAPH_Y -LA("e-") -end END 54
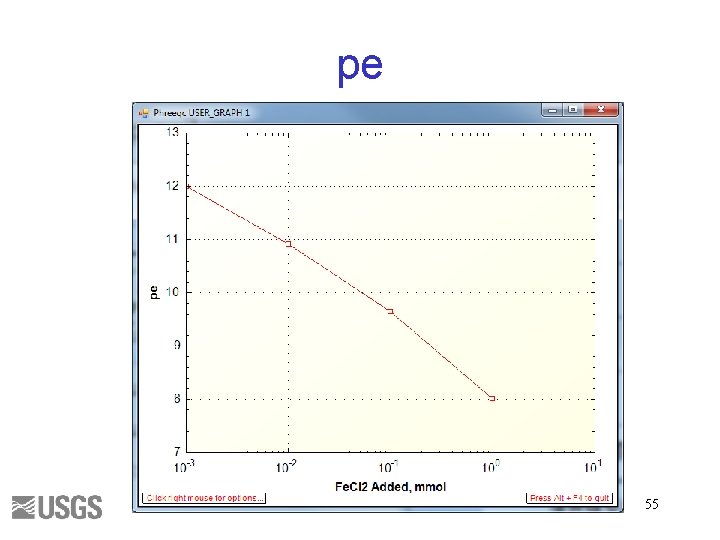
pe 55
![What is pe Fe2 Fe3 epe log Fe3Fe2 13 What is pe? Fe+2 = Fe+3 + epe = log( [Fe+3]/[Fe+2] ) + 13](https://slidetodoc.com/presentation_image/200b8de3ad78ea91e8cd99b28236b61f/image-56.jpg)
What is pe? Fe+2 = Fe+3 + epe = log( [Fe+3]/[Fe+2] ) + 13 HS- + 4 H 2 O = SO 4 -2 + 9 H+ + 8 epe = log( [SO 4 -2]/[HS-] ) – 9/8 p. H + 4. 21 N 2 + 6 H 2 O = 2 NO 3 - + 12 H+ + 10 epe = 0. 1 log( [NO 3 -]2/[N 2] ) – 1. 2 p. H + 20. 7 pe = 16. 9 Eh, Eh in volts (platinum electrode measurement) 56
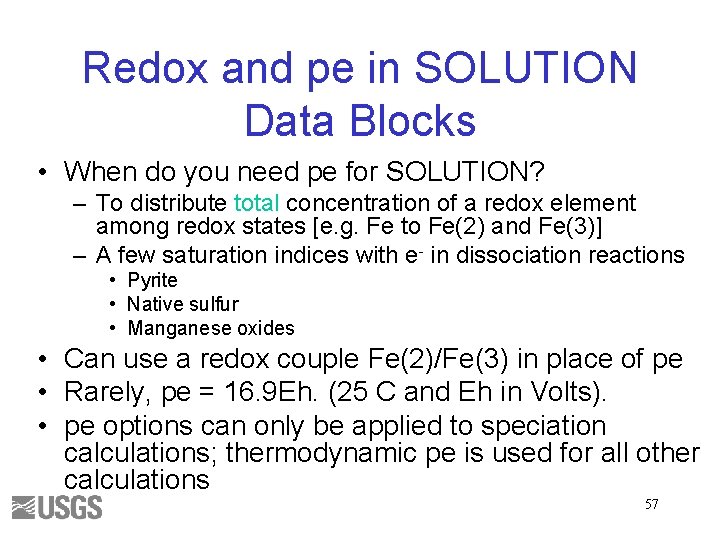
Redox and pe in SOLUTION Data Blocks • When do you need pe for SOLUTION? – To distribute total concentration of a redox element among redox states [e. g. Fe to Fe(2) and Fe(3)] – A few saturation indices with e- in dissociation reactions • Pyrite • Native sulfur • Manganese oxides • Can use a redox couple Fe(2)/Fe(3) in place of pe • Rarely, pe = 16. 9 Eh. (25 C and Eh in Volts). • pe options can only be applied to speciation calculations; thermodynamic pe is used for all other calculations 57
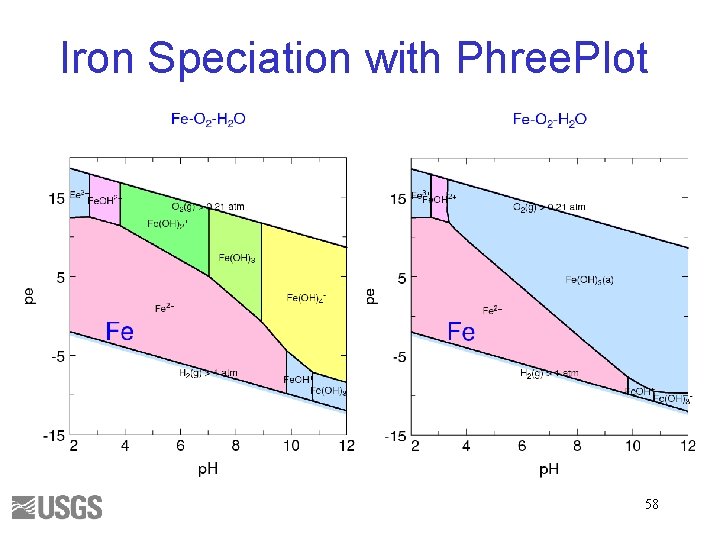
Iron Speciation with Phree. Plot 58
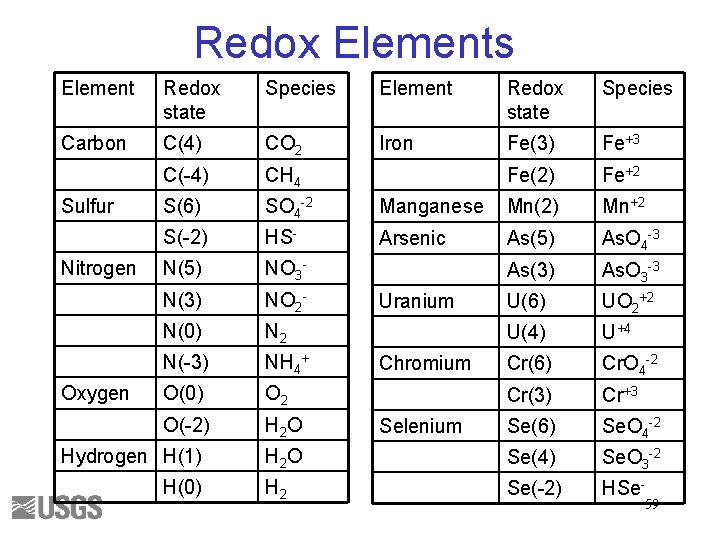
Redox Elements Element Redox state Species Carbon C(4) CO 2 Iron Fe(3) Fe+3 C(-4) CH 4 Fe(2) Fe+2 S(6) SO 4 -2 Manganese Mn(2) Mn+2 S(-2) HS- Arsenic As(5) As. O 4 -3 N(5) NO 3 - As(3) As. O 3 -3 N(3) NO 2 - U(6) UO 2+2 N(0) N 2 U(4) U+4 N(-3) NH 4+ Cr(6) Cr. O 4 -2 O(0) O 2 Cr(3) Cr+3 O(-2) H 2 O Se(6) Se. O 4 -2 H 2 O Se(4) Se. O 3 -2 H 2 Se(-2) HSe- Sulfur Nitrogen Oxygen Hydrogen H(1) H(0) Uranium Chromium Selenium 59
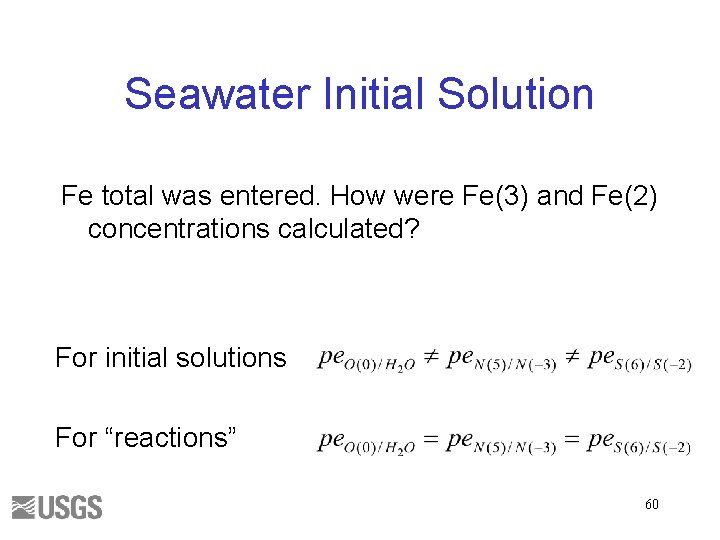
Seawater Initial Solution Fe total was entered. How were Fe(3) and Fe(2) concentrations calculated? For initial solutions For “reactions” 60
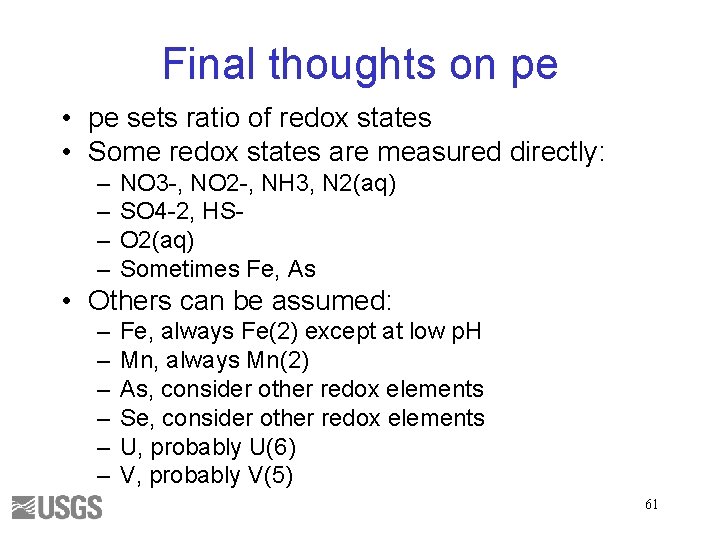
Final thoughts on pe • pe sets ratio of redox states • Some redox states are measured directly: – – NO 3 -, NO 2 -, NH 3, N 2(aq) SO 4 -2, HSO 2(aq) Sometimes Fe, As • Others can be assumed: – – – Fe, always Fe(2) except at low p. H Mn, always Mn(2) As, consider other redox elements Se, consider other redox elements U, probably U(6) V, probably V(5) 61
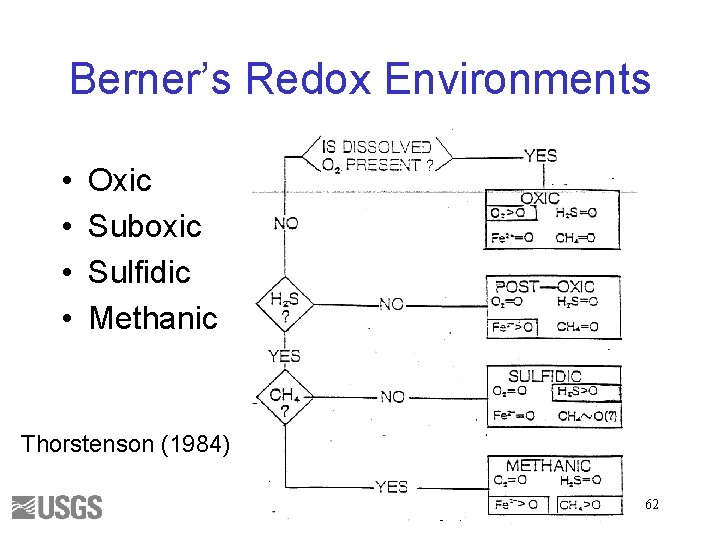
Berner’s Redox Environments • • Oxic Suboxic Sulfidic Methanic Thorstenson (1984) 62
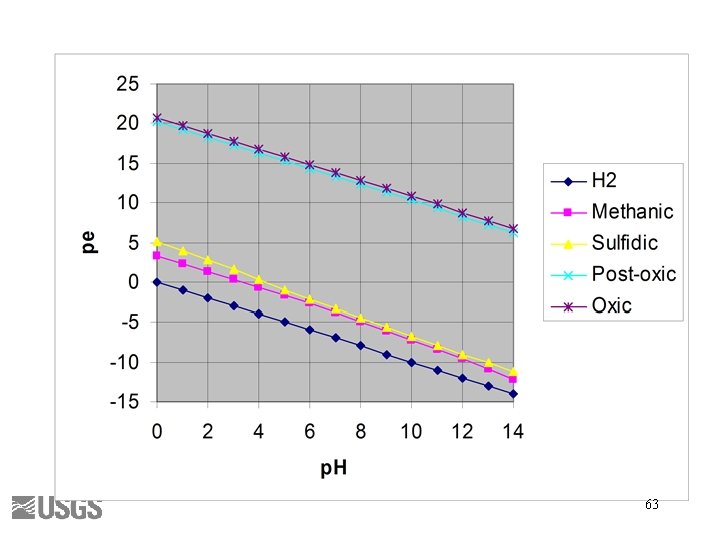
63
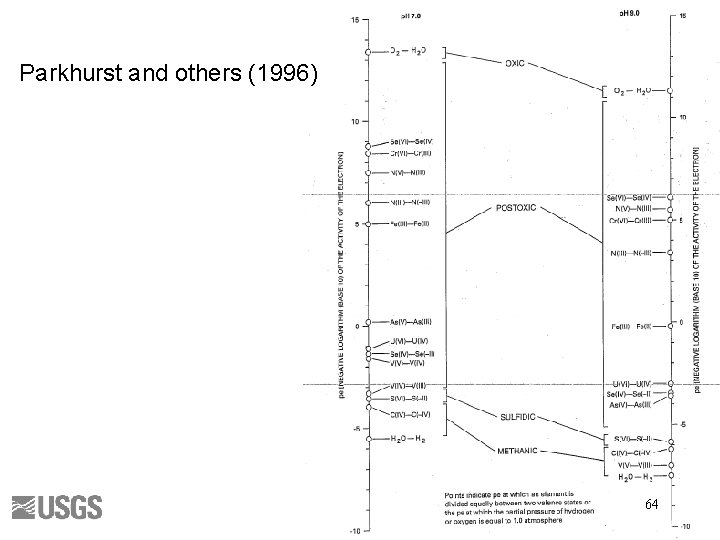
Parkhurst and others (1996) 64
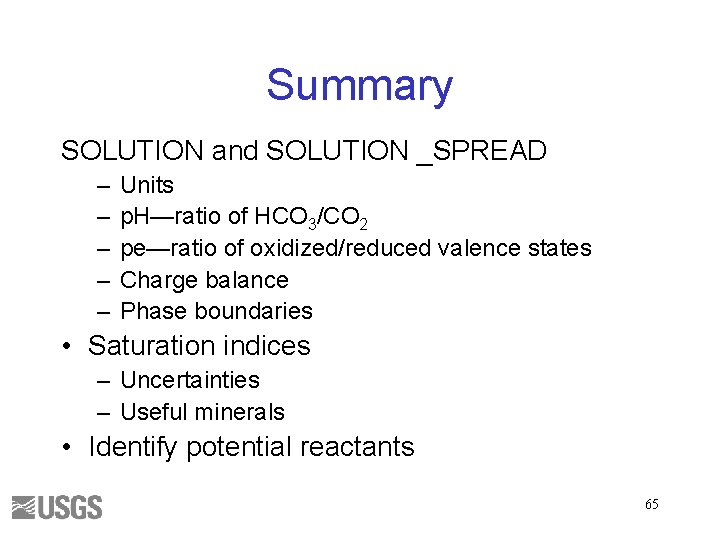
Summary SOLUTION and SOLUTION _SPREAD – – – Units p. H—ratio of HCO 3/CO 2 pe—ratio of oxidized/reduced valence states Charge balance Phase boundaries • Saturation indices – Uncertainties – Useful minerals • Identify potential reactants 65
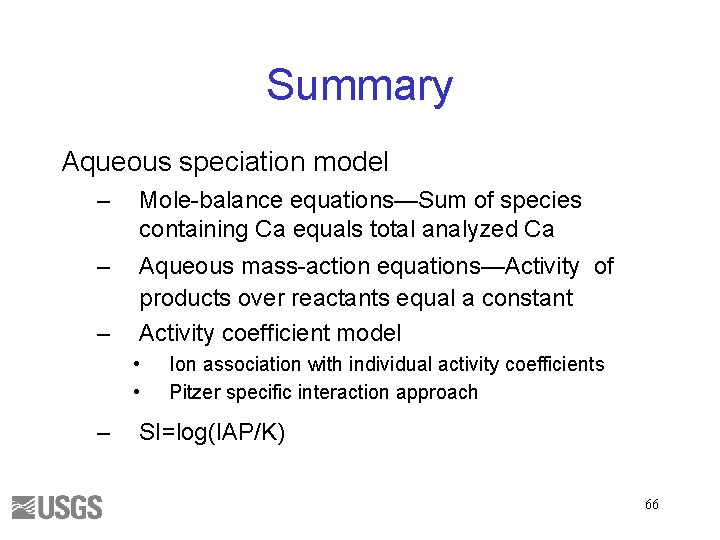
Summary Aqueous speciation model – Mole-balance equations—Sum of species containing Ca equals total analyzed Ca – Aqueous mass-action equations—Activity of products over reactants equal a constant Activity coefficient model – • • – Ion association with individual activity coefficients Pitzer specific interaction approach SI=log(IAP/K) 66
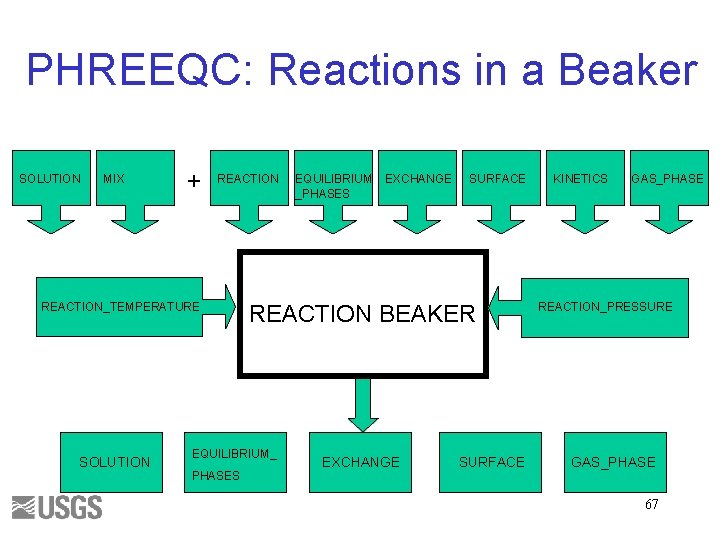
PHREEQC: Reactions in a Beaker SOLUTION MIX + REACTION_TEMPERATURE SOLUTION EXCHANGE SURFACE REACTION BEAKER EQUILIBRIUM_ PHASES EQUILIBRIUM _PHASES EXCHANGE SURFACE KINETICS GAS_PHASE REACTION_PRESSURE GAS_PHASE 67
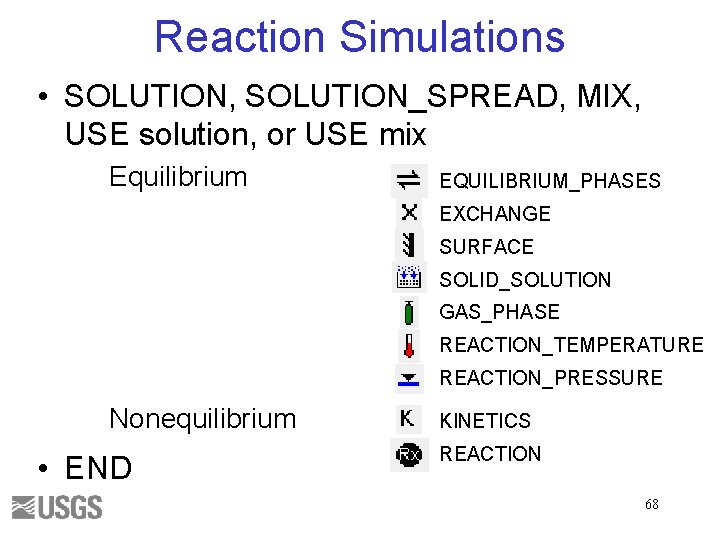
Reaction Simulations • SOLUTION, SOLUTION_SPREAD, MIX, USE solution, or USE mix Equilibrium EQUILIBRIUM_PHASES EXCHANGE SURFACE SOLID_SOLUTION GAS_PHASE REACTION_TEMPERATURE REACTION_PRESSURE Nonequilibrium • END KINETICS REACTION 68
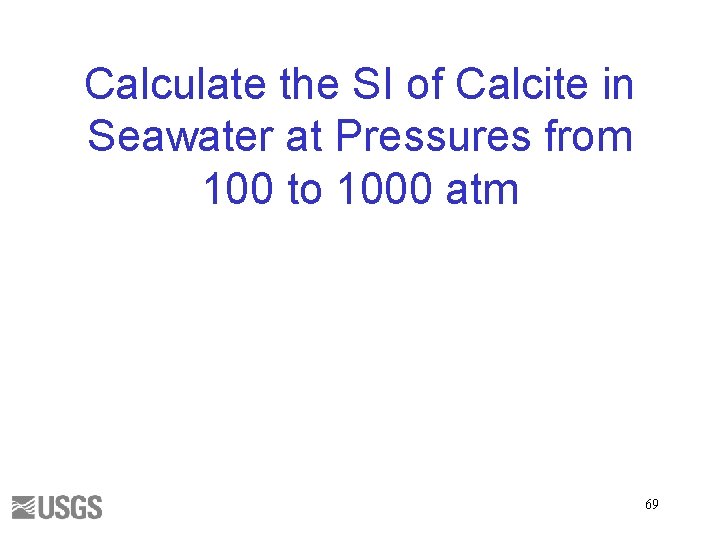
Calculate the SI of Calcite in Seawater at Pressures from 100 to 1000 atm 69
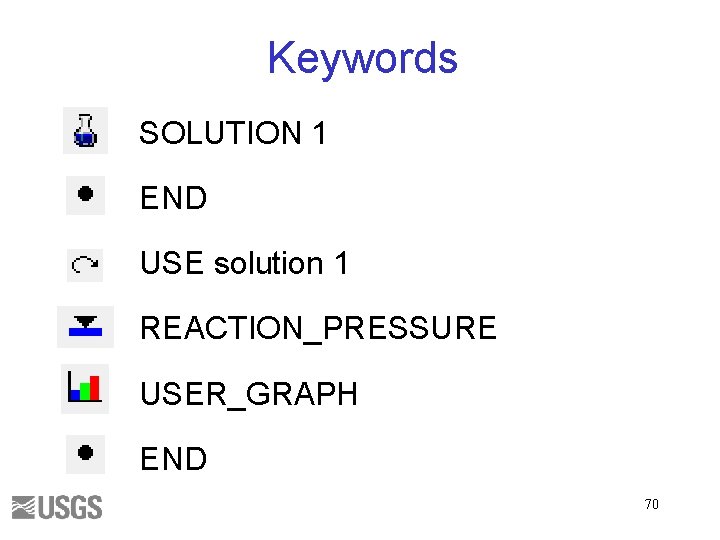
Keywords SOLUTION 1 END USE solution 1 REACTION_PRESSURE USER_GRAPH END 70
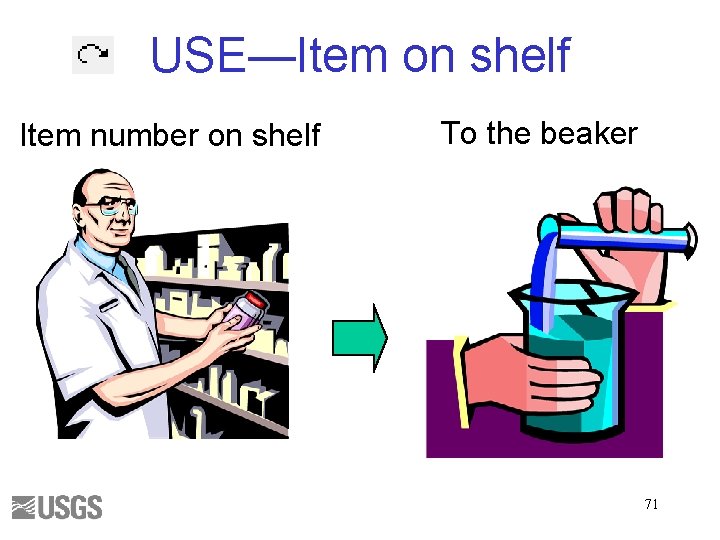
USE—Item on shelf Item number on shelf To the beaker 71
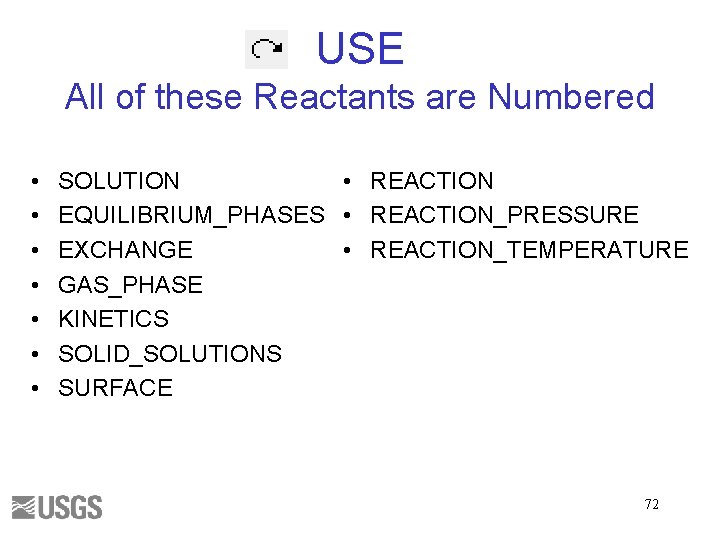
USE All of these Reactants are Numbered • • SOLUTION • REACTION EQUILIBRIUM_PHASES • REACTION_PRESSURE EXCHANGE • REACTION_TEMPERATURE GAS_PHASE KINETICS SOLID_SOLUTIONS SURFACE 72
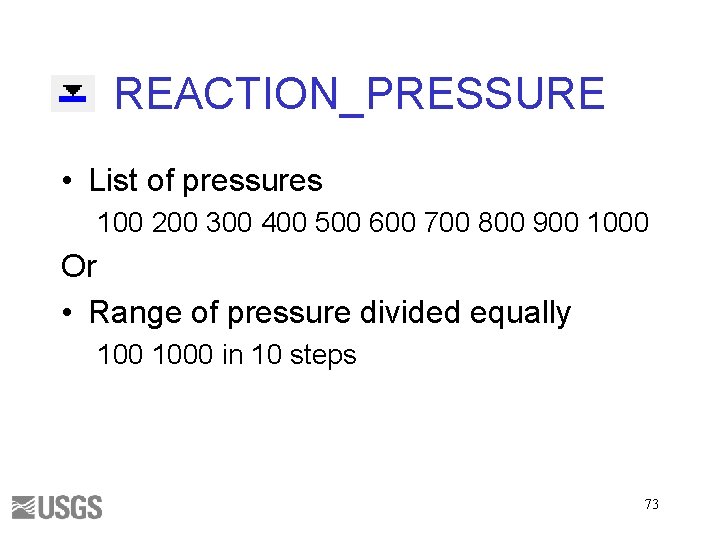
REACTION_PRESSURE • List of pressures 100 200 300 400 500 600 700 800 900 1000 Or • Range of pressure divided equally 1000 in 10 steps 73
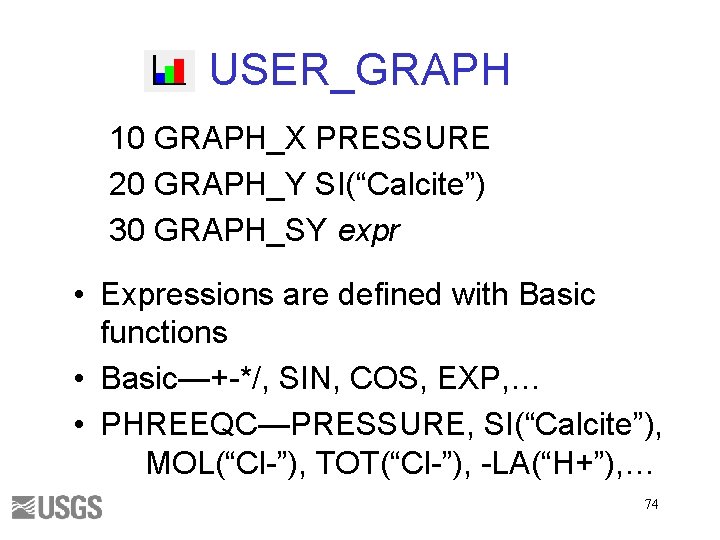
USER_GRAPH 10 GRAPH_X PRESSURE 20 GRAPH_Y SI(“Calcite”) 30 GRAPH_SY expr • Expressions are defined with Basic functions • Basic—+-*/, SIN, COS, EXP, … • PHREEQC—PRESSURE, SI(“Calcite”), MOL(“Cl-”), TOT(“Cl-”), -LA(“H+”), … 74
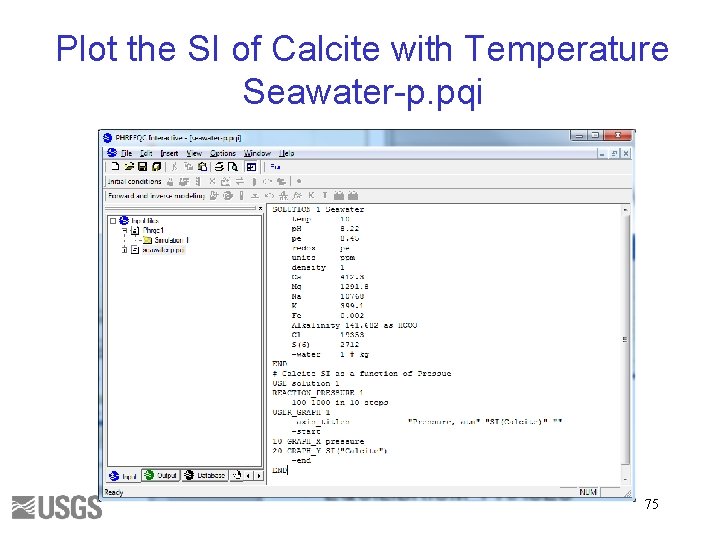
Plot the SI of Calcite with Temperature Seawater-p. pqi 75
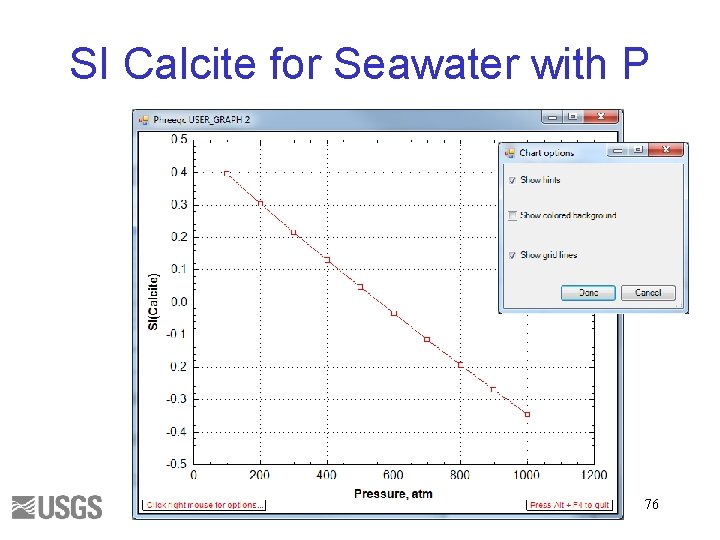
SI Calcite for Seawater with P 76
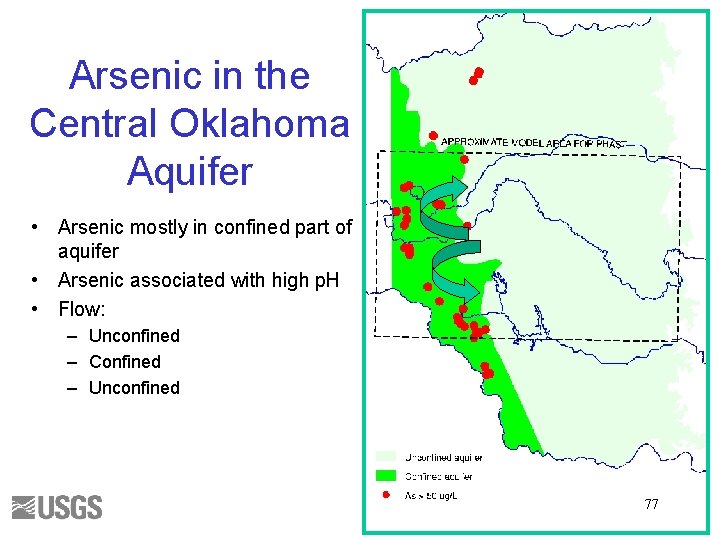
Arsenic in the Central Oklahoma Aquifer • Arsenic mostly in confined part of aquifer • Arsenic associated with high p. H • Flow: – Unconfined – Confined – Unconfined 77
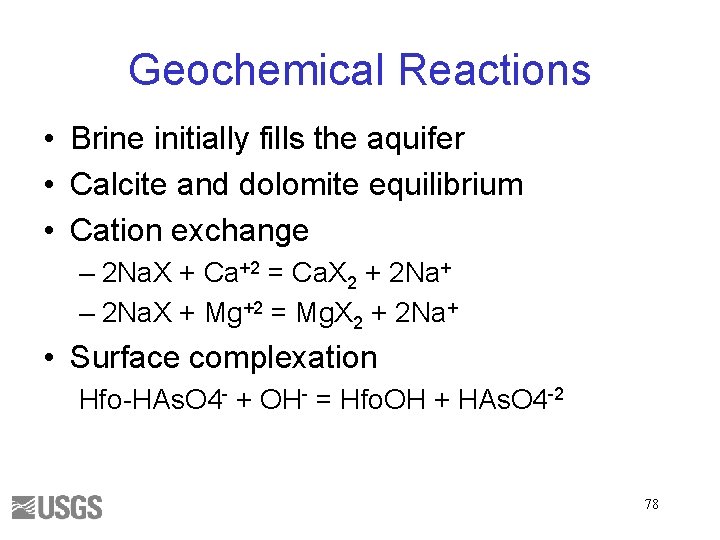
Geochemical Reactions • Brine initially fills the aquifer • Calcite and dolomite equilibrium • Cation exchange – 2 Na. X + Ca+2 = Ca. X 2 + 2 Na+ – 2 Na. X + Mg+2 = Mg. X 2 + 2 Na+ • Surface complexation Hfo-HAs. O 4 - + OH- = Hfo. OH + HAs. O 4 -2 78
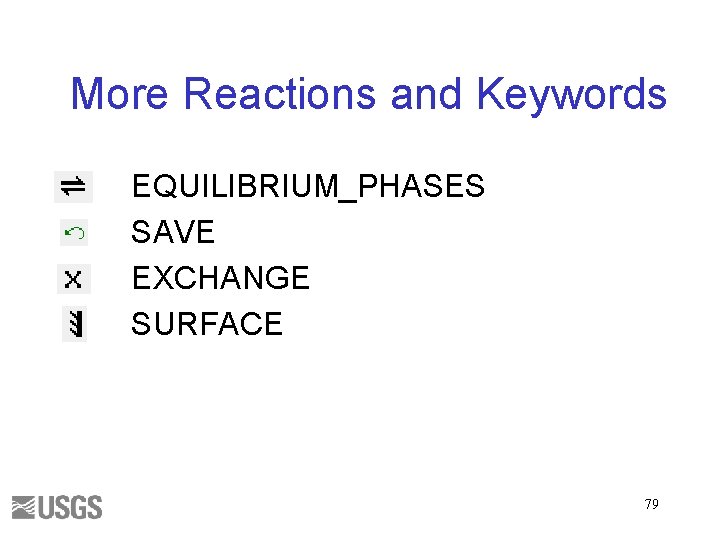
More Reactions and Keywords EQUILIBRIUM_PHASES SAVE EXCHANGE SURFACE 79
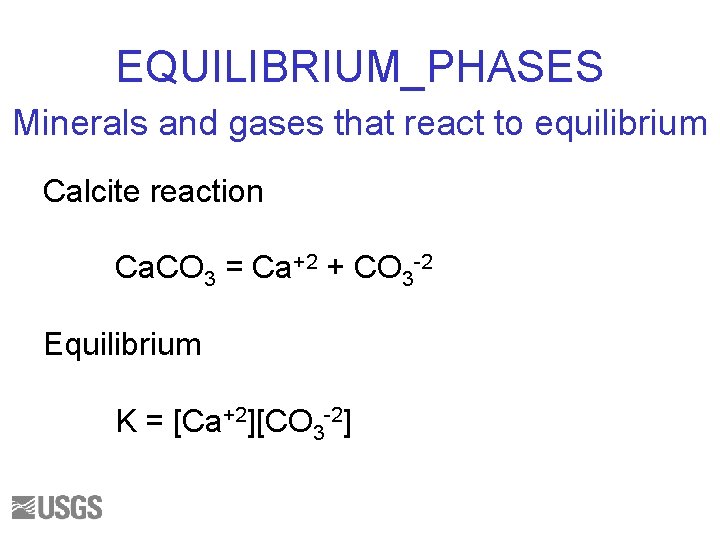
EQUILIBRIUM_PHASES Minerals and gases that react to equilibrium Calcite reaction Ca. CO 3 = Ca+2 + CO 3 -2 Equilibrium K = [Ca+2][CO 3 -2]
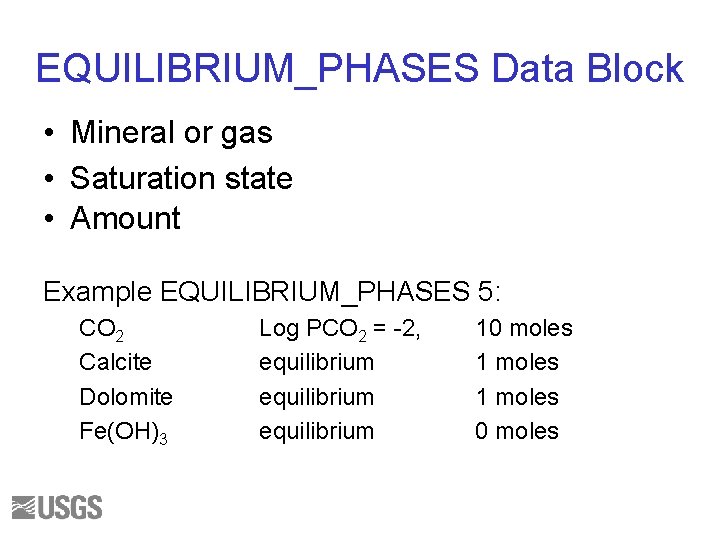
EQUILIBRIUM_PHASES Data Block • Mineral or gas • Saturation state • Amount Example EQUILIBRIUM_PHASES 5: CO 2 Calcite Dolomite Fe(OH)3 Log PCO 2 = -2, equilibrium 10 moles 1 moles 0 moles
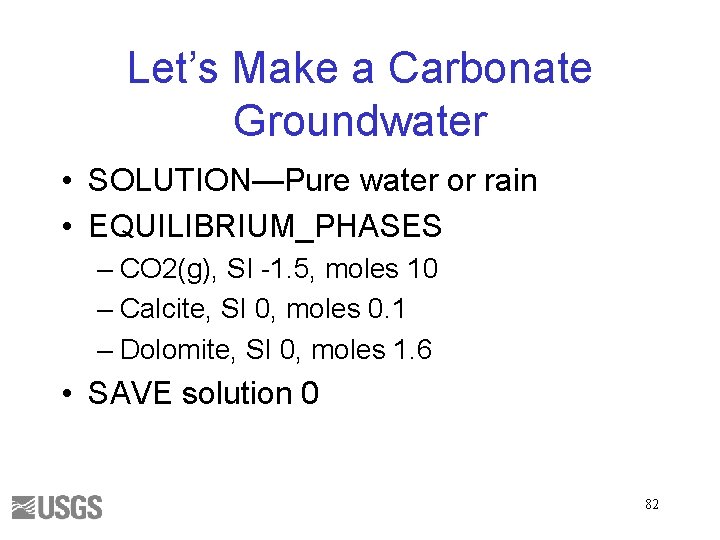
Let’s Make a Carbonate Groundwater • SOLUTION—Pure water or rain • EQUILIBRIUM_PHASES – CO 2(g), SI -1. 5, moles 10 – Calcite, SI 0, moles 0. 1 – Dolomite, SI 0, moles 1. 6 • SAVE solution 0 82
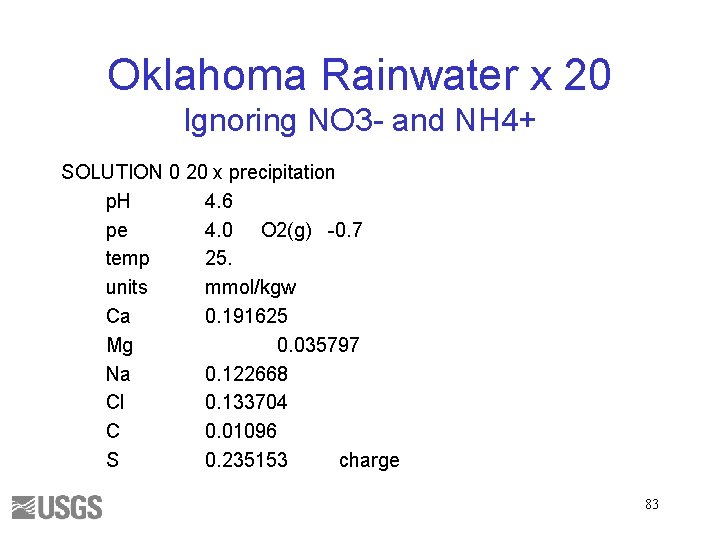
Oklahoma Rainwater x 20 Ignoring NO 3 - and NH 4+ SOLUTION 0 20 x precipitation p. H 4. 6 pe 4. 0 O 2(g) -0. 7 temp 25. units mmol/kgw Ca 0. 191625 Mg 0. 035797 Na 0. 122668 Cl 0. 133704 C 0. 01096 S 0. 235153 charge 83
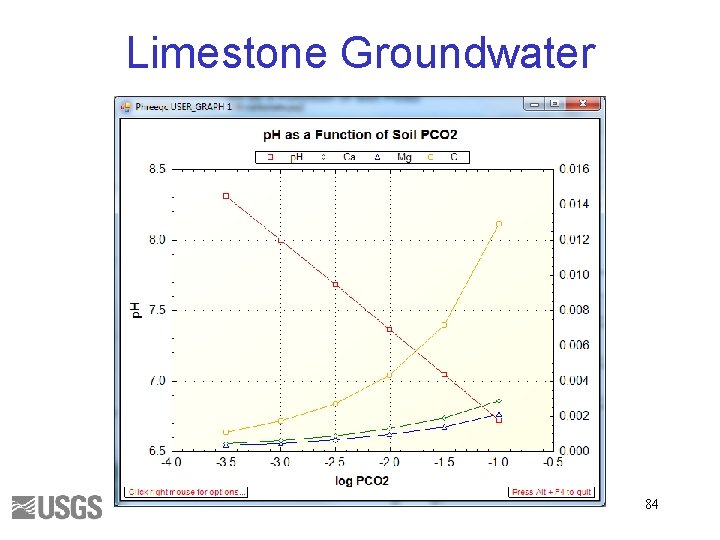
Limestone Groundwater 84
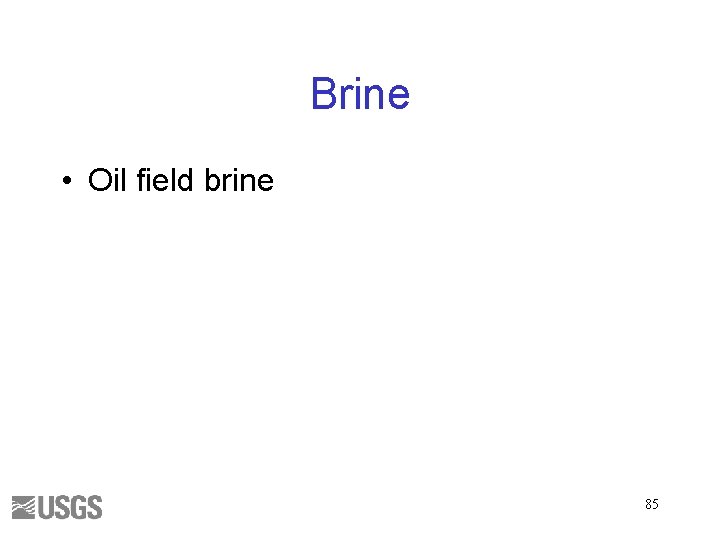
Brine • Oil field brine 85
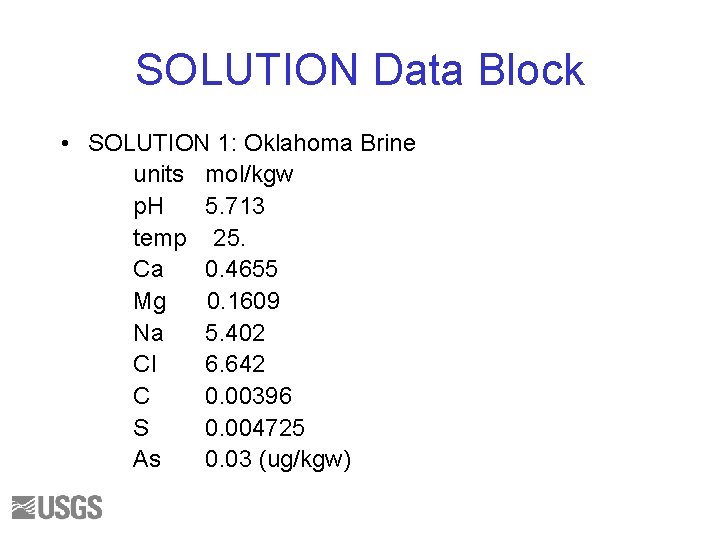
SOLUTION Data Block • SOLUTION 1: Oklahoma Brine units mol/kgw p. H 5. 713 temp 25. Ca 0. 4655 Mg 0. 1609 Na 5. 402 Cl 6. 642 C 0. 00396 S 0. 004725 As 0. 03 (ug/kgw)
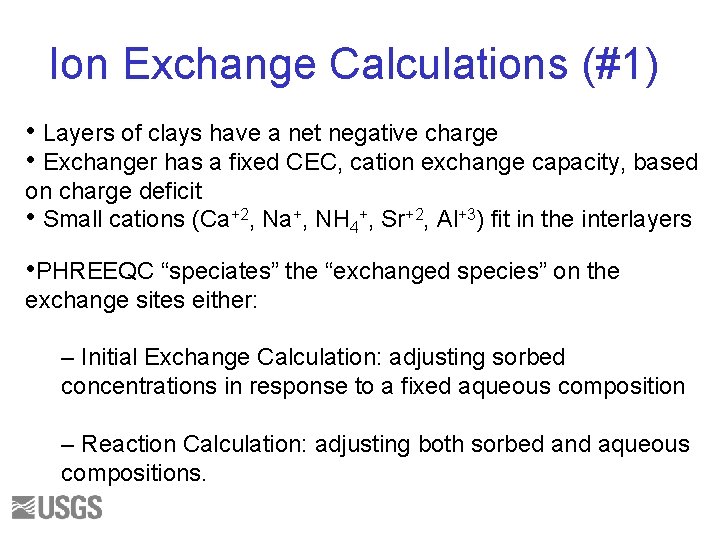
Ion Exchange Calculations (#1) • Layers of clays have a net negative charge • Exchanger has a fixed CEC, cation exchange capacity, based on charge deficit • Small cations (Ca+2, Na+, NH 4+, Sr+2, Al+3) fit in the interlayers • PHREEQC “speciates” the “exchanged species” on the exchange sites either: – Initial Exchange Calculation: adjusting sorbed concentrations in response to a fixed aqueous composition – Reaction Calculation: adjusting both sorbed and aqueous compositions.
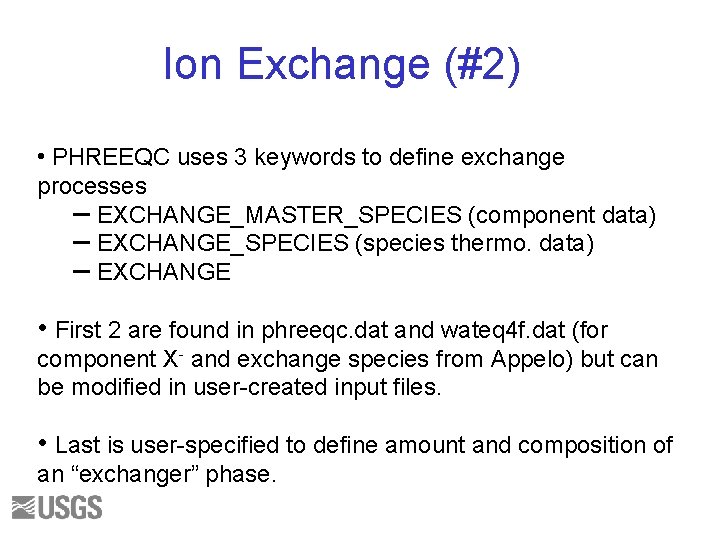
Ion Exchange (#2) • PHREEQC uses 3 keywords to define exchange processes – EXCHANGE_MASTER_SPECIES (component data) – EXCHANGE_SPECIES (species thermo. data) – EXCHANGE • First 2 are found in phreeqc. dat and wateq 4 f. dat (for component X- and exchange species from Appelo) but can be modified in user-created input files. • Last is user-specified to define amount and composition of an “exchanger” phase.
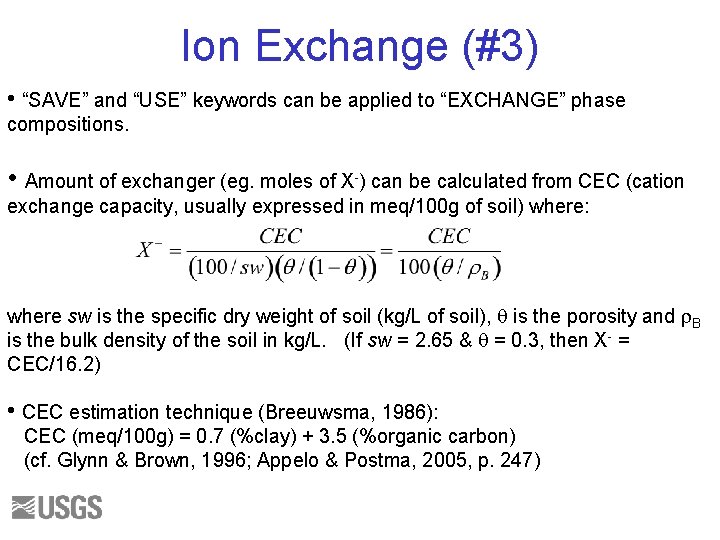
Ion Exchange (#3) • “SAVE” and “USE” keywords can be applied to “EXCHANGE” phase compositions. • Amount of exchanger (eg. moles of X-) can be calculated from CEC (cation exchange capacity, usually expressed in meq/100 g of soil) where: where sw is the specific dry weight of soil (kg/L of soil), q is the porosity and r. B is the bulk density of the soil in kg/L. (If sw = 2. 65 & q = 0. 3, then X- = CEC/16. 2) • CEC estimation technique (Breeuwsma, 1986): CEC (meq/100 g) = 0. 7 (%clay) + 3. 5 (%organic carbon) (cf. Glynn & Brown, 1996; Appelo & Postma, 2005, p. 247)
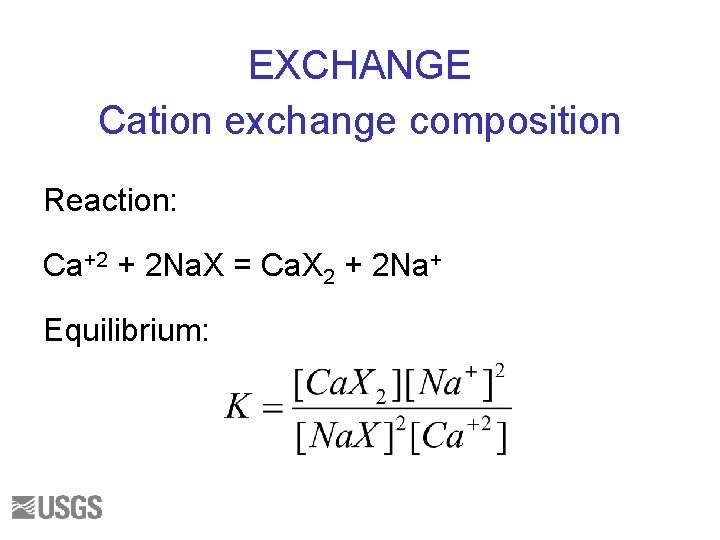
EXCHANGE Cation exchange composition Reaction: Ca+2 + 2 Na. X = Ca. X 2 + 2 Na+ Equilibrium:
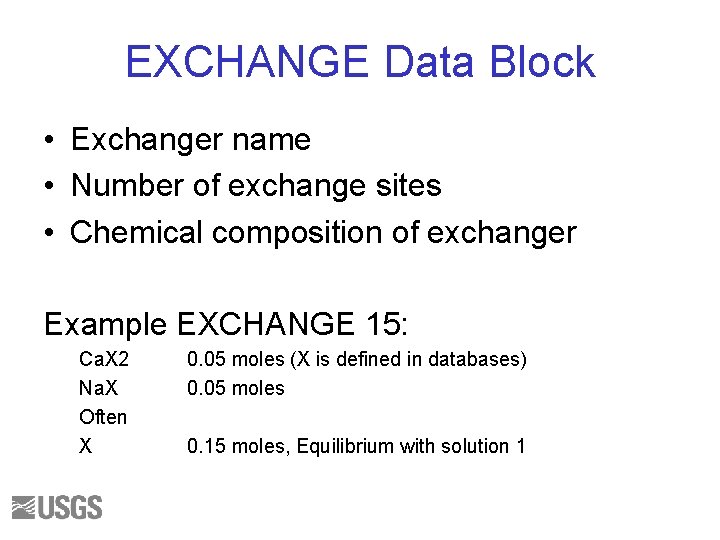
EXCHANGE Data Block • Exchanger name • Number of exchange sites • Chemical composition of exchanger Example EXCHANGE 15: Ca. X 2 Na. X Often X 0. 05 moles (X is defined in databases) 0. 05 moles 0. 15 moles, Equilibrium with solution 1
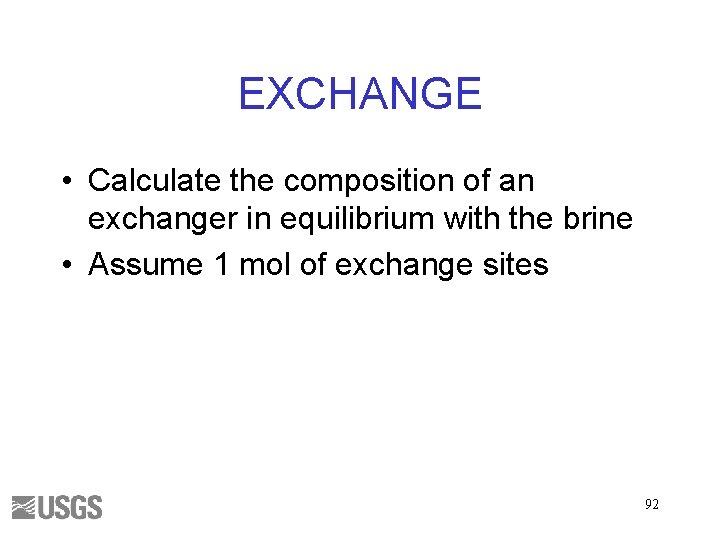
EXCHANGE • Calculate the composition of an exchanger in equilibrium with the brine • Assume 1 mol of exchange sites 92
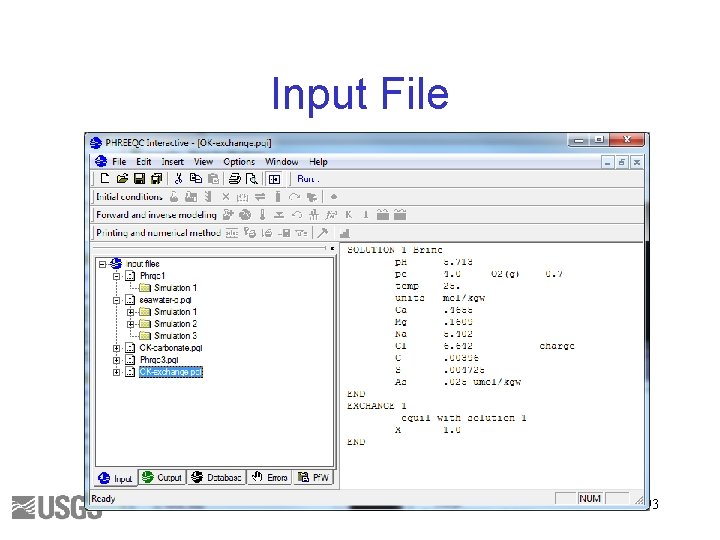
Input File 93
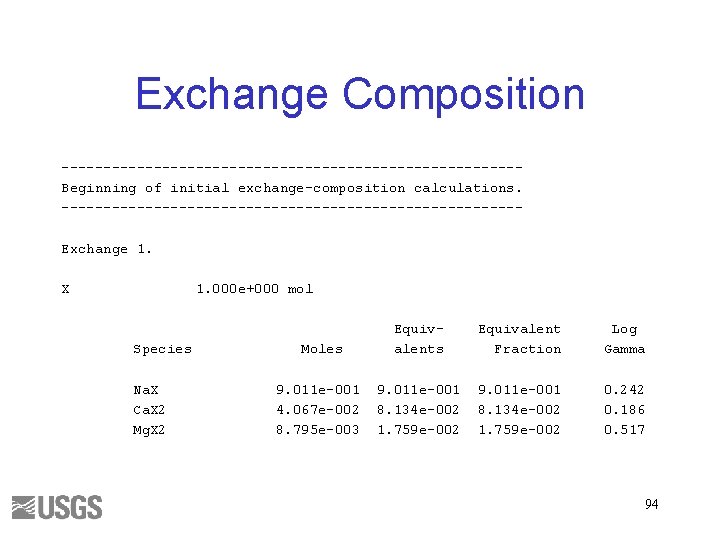
Exchange Composition ---------------------------Beginning of initial exchange-composition calculations. ---------------------------Exchange 1. X 1. 000 e+000 mol Species Na. X Ca. X 2 Mg. X 2 Moles Equivalent Fraction Log Gamma 9. 011 e-001 4. 067 e-002 8. 795 e-003 9. 011 e-001 8. 134 e-002 1. 759 e-002 0. 242 0. 186 0. 517 94
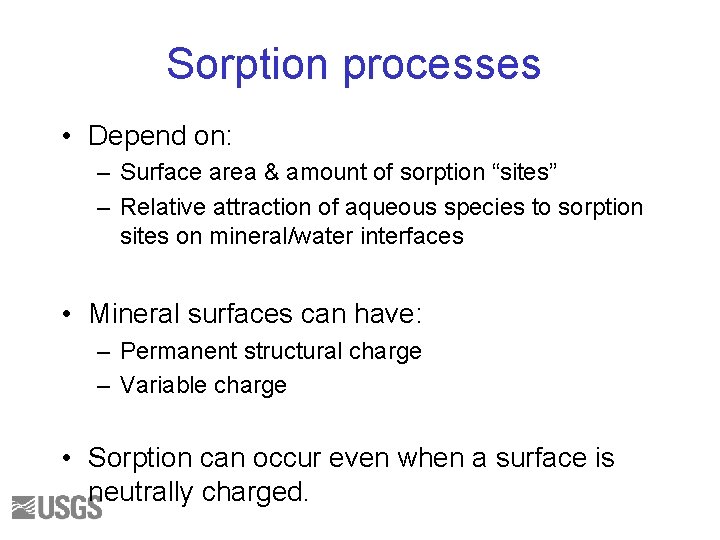
Sorption processes • Depend on: – Surface area & amount of sorption “sites” – Relative attraction of aqueous species to sorption sites on mineral/water interfaces • Mineral surfaces can have: – Permanent structural charge – Variable charge • Sorption can occur even when a surface is neutrally charged.
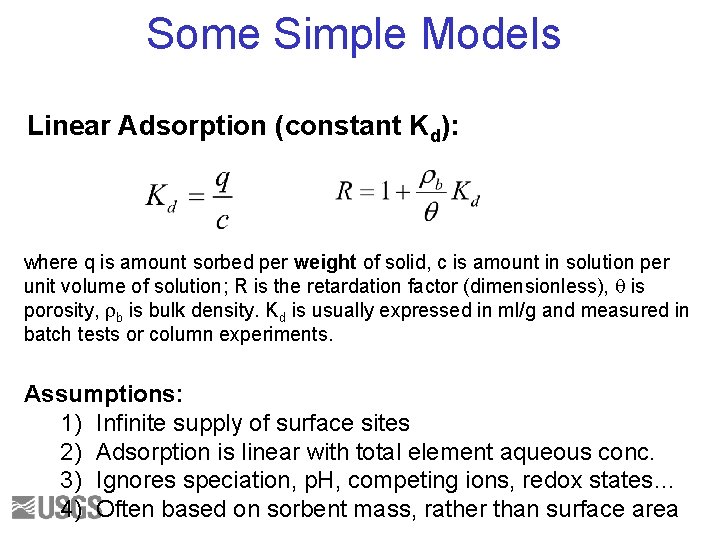
Some Simple Models Linear Adsorption (constant Kd): where q is amount sorbed per weight of solid, c is amount in solution per unit volume of solution; R is the retardation factor (dimensionless), q is porosity, rb is bulk density. Kd is usually expressed in ml/g and measured in batch tests or column experiments. Assumptions: 1) Infinite supply of surface sites 2) Adsorption is linear with total element aqueous conc. 3) Ignores speciation, p. H, competing ions, redox states… 4) Often based on sorbent mass, rather than surface area
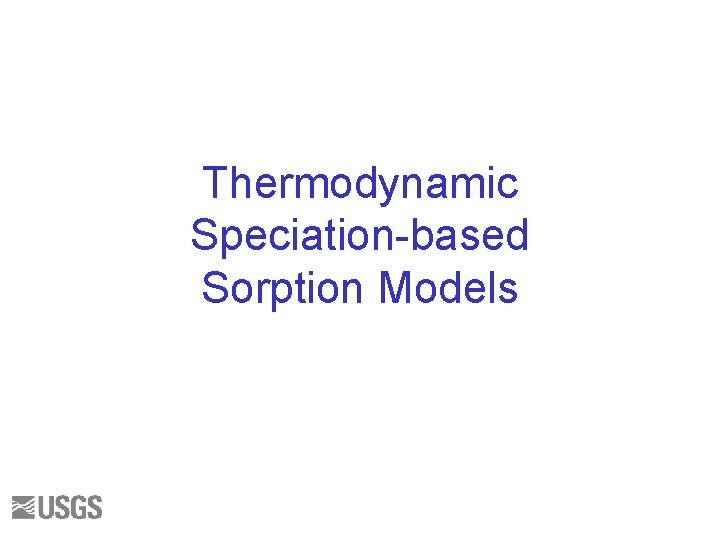
Thermodynamic Speciation-based Sorption Models
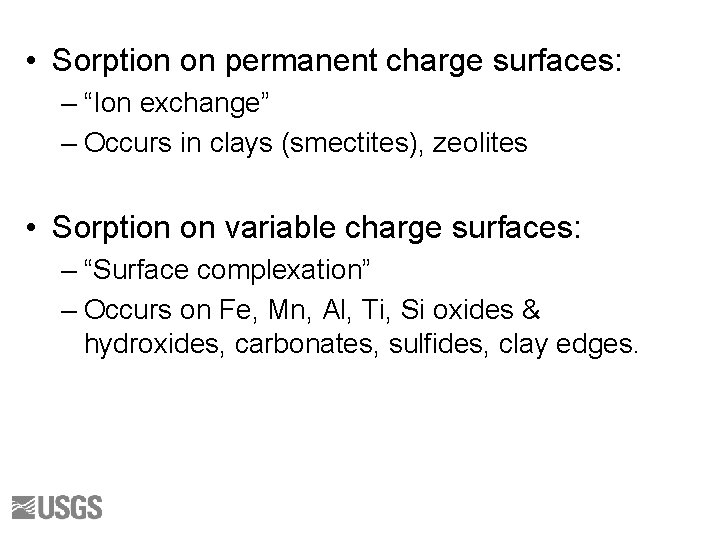
• Sorption on permanent charge surfaces: – “Ion exchange” – Occurs in clays (smectites), zeolites • Sorption on variable charge surfaces: – “Surface complexation” – Occurs on Fe, Mn, Al, Ti, Si oxides & hydroxides, carbonates, sulfides, clay edges.
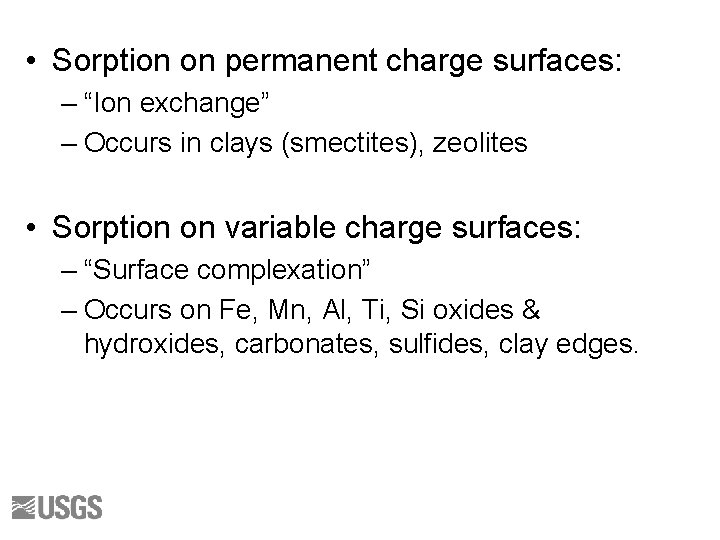
• Sorption on permanent charge surfaces: – “Ion exchange” – Occurs in clays (smectites), zeolites • Sorption on variable charge surfaces: – “Surface complexation” – Occurs on Fe, Mn, Al, Ti, Si oxides & hydroxides, carbonates, sulfides, clay edges.
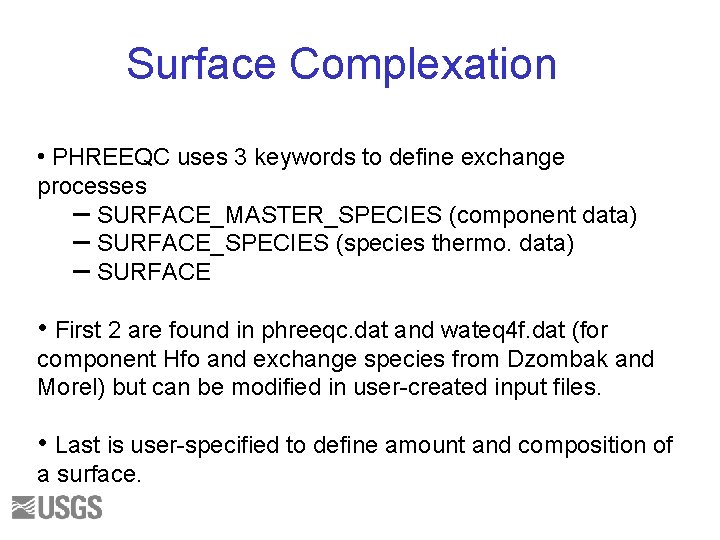
Surface Complexation • PHREEQC uses 3 keywords to define exchange processes – SURFACE_MASTER_SPECIES (component data) – SURFACE_SPECIES (species thermo. data) – SURFACE • First 2 are found in phreeqc. dat and wateq 4 f. dat (for component Hfo and exchange species from Dzombak and Morel) but can be modified in user-created input files. • Last is user-specified to define amount and composition of a surface.
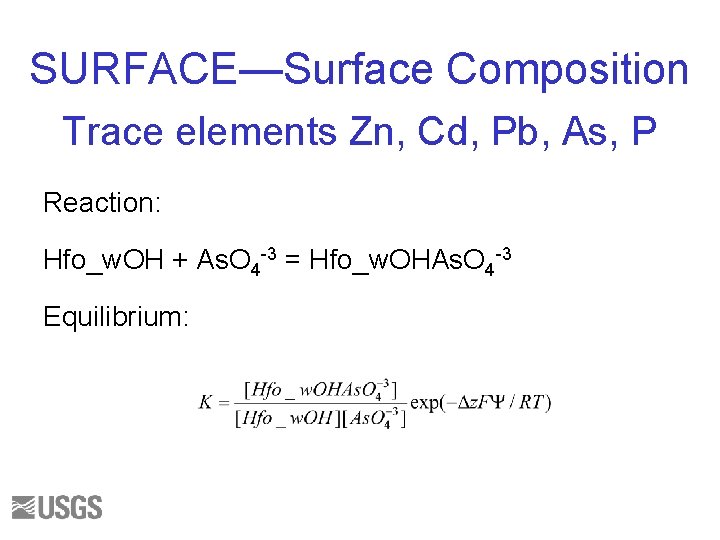
SURFACE—Surface Composition Trace elements Zn, Cd, Pb, As, P Reaction: Hfo_w. OH + As. O 4 -3 = Hfo_w. OHAs. O 4 -3 Equilibrium:
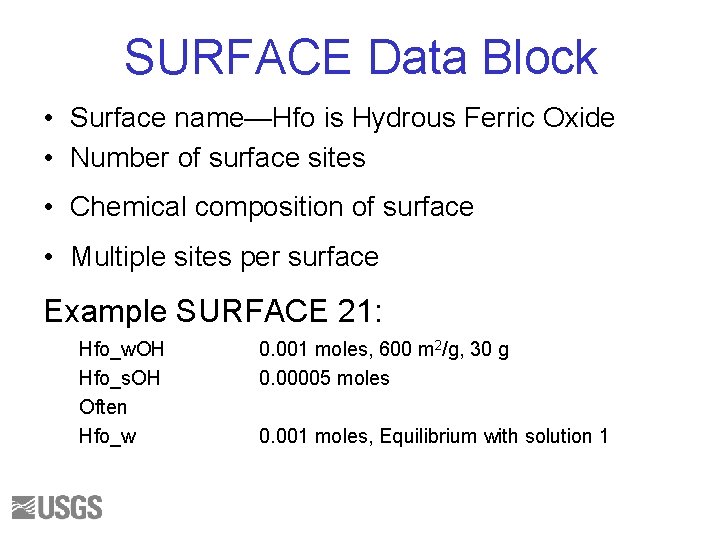
SURFACE Data Block • Surface name—Hfo is Hydrous Ferric Oxide • Number of surface sites • Chemical composition of surface • Multiple sites per surface Example SURFACE 21: Hfo_w. OH Hfo_s. OH Often Hfo_w 0. 001 moles, 600 m 2/g, 30 g 0. 00005 moles 0. 001 moles, Equilibrium with solution 1
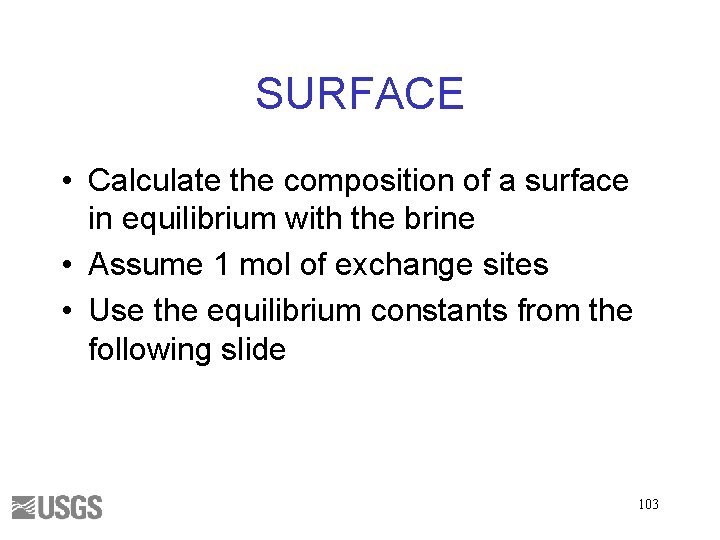
SURFACE • Calculate the composition of a surface in equilibrium with the brine • Assume 1 mol of exchange sites • Use the equilibrium constants from the following slide 103
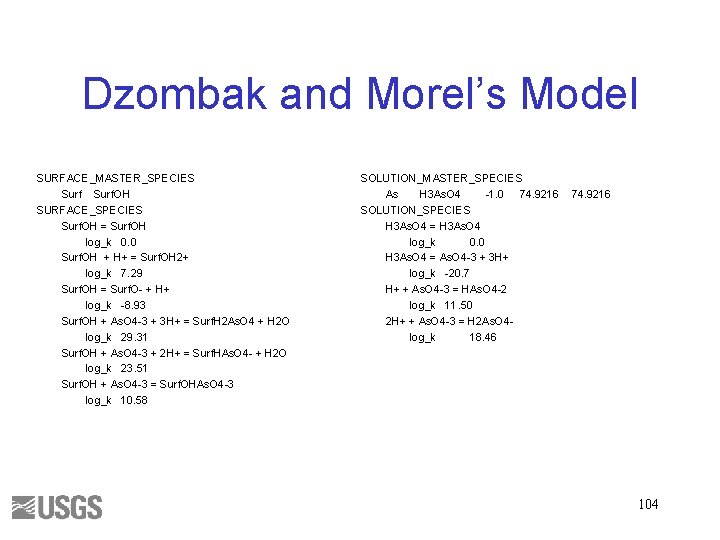
Dzombak and Morel’s Model SURFACE_MASTER_SPECIES Surf. OH SURFACE_SPECIES Surf. OH = Surf. OH log_k 0. 0 Surf. OH + H+ = Surf. OH 2+ log_k 7. 29 Surf. OH = Surf. O- + H+ log_k -8. 93 Surf. OH + As. O 4 -3 + 3 H+ = Surf. H 2 As. O 4 + H 2 O log_k 29. 31 Surf. OH + As. O 4 -3 + 2 H+ = Surf. HAs. O 4 - + H 2 O log_k 23. 51 Surf. OH + As. O 4 -3 = Surf. OHAs. O 4 -3 log_k 10. 58 SOLUTION_MASTER_SPECIES As H 3 As. O 4 -1. 0 74. 9216 SOLUTION_SPECIES H 3 As. O 4 = H 3 As. O 4 log_k 0. 0 H 3 As. O 4 = As. O 4 -3 + 3 H+ log_k -20. 7 H+ + As. O 4 -3 = HAs. O 4 -2 log_k 11. 50 2 H+ + As. O 4 -3 = H 2 As. O 4 log_k 18. 46 74. 9216 104
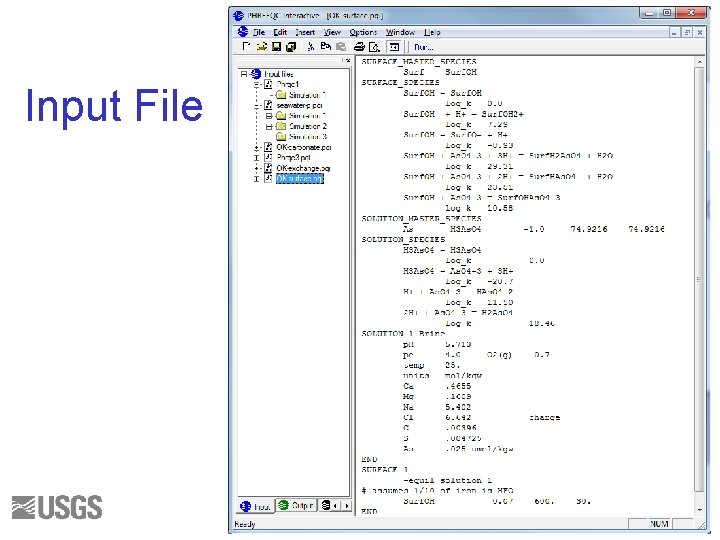
Input File 105
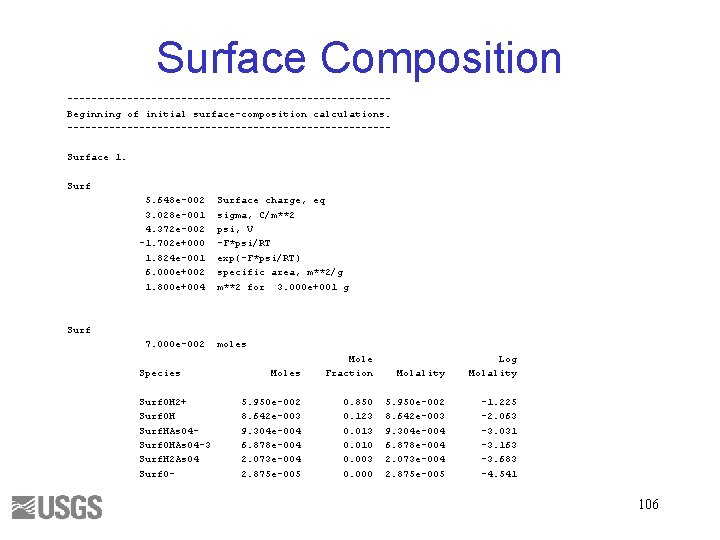
Surface Composition ---------------------------Beginning of initial surface-composition calculations. ---------------------------Surface 1. Surf 5. 648 e-002 3. 028 e-001 4. 372 e-002 -1. 702 e+000 1. 824 e-001 6. 000 e+002 1. 800 e+004 Surface charge, eq sigma, C/m**2 psi, V -F*psi/RT exp(-F*psi/RT) specific area, m**2/g m**2 for 3. 000 e+001 g Surf 7. 000 e-002 Species Surf. OH 2+ Surf. OH Surf. HAs. O 4 Surf. OHAs. O 4 -3 Surf. H 2 As. O 4 Surf. O- moles Mole Fraction Molality Log Molality 5. 950 e-002 8. 642 e-003 9. 304 e-004 6. 878 e-004 2. 073 e-004 2. 875 e-005 0. 850 0. 123 0. 010 0. 003 0. 000 5. 950 e-002 8. 642 e-003 9. 304 e-004 6. 878 e-004 2. 073 e-004 2. 875 e-005 -1. 225 -2. 063 -3. 031 -3. 163 -3. 683 -4. 541 106
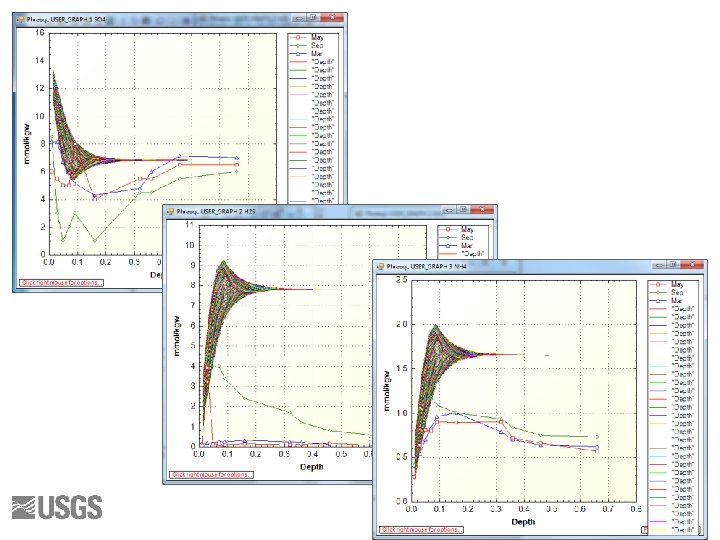
107
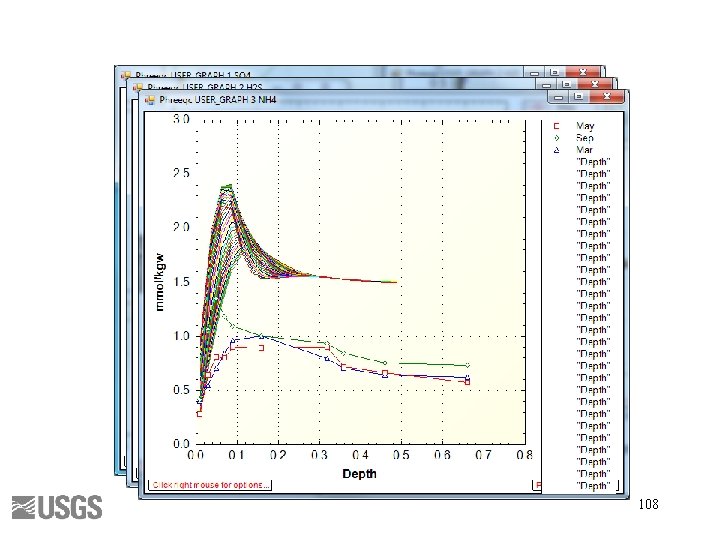
108
Markus pitzer
Dr michael pitzer
Michael pitzer
Dan pitzer
Reactions in aqueous solutions
Balancing redox reactions in basic solution
Chapter 13 ions in aqueous solutions
Aqueous solutions module
Chemical reactions section 3 reactions in aqueous solutions
Freezing point chapter 13
General properties of aqueous solutions
Reactions in aqueous solutions practice problems
Electrical conductivity of aqueous solutions
Chapter 4 reactions in aqueous solutions
Are all aqueous solutions homogeneous
Are aqueous solutions homogeneous mixtures
Solubility guidelines for aqueous solutions
Concentrated solution
Chapter 4 reactions in aqueous solutions worksheet answers
Dilute solution
C6h12 fuerza intermolecular
Dipolo dipolo inducido
Uniones intermoleculares
Ion dipole intermolecular forces
Dr tatiana erukhimova
Hittorf
Types of reaction in non aqueous solvents
For clarity of aqueous extract test container autoclaved at
Contoh pelarut non air
What are protonic and non protonic solvents
Abbreviation for solid in chemistry
Additional aspects of aqueous equilibria
Chapter 15 water and aqueous systems worksheet answers
Chapter 15 water and aqueous systems
Do pickles conduct electricity
Additional aspects of aqueous equilibria
Aqueous reactions and solution stoichiometry
An artificial cell consisting of an aqueous solution
Aqueous acid base titration
Heterogeneous aqueous systems
Chapter 15 water and aqueous systems
Is water aqueous
Types of reaction in non aqueous solvents
Syneresis impression material
Advantages and disadvantages of addition silicone
Reactions of alkenes 2 chemsheets
Aqueous equilibria
Knockhardy chemistry
Aqueous ionic equilibrium
Acid base concept in non aqueous media
Non aqueous media
Birdshot retinochoroidopathy
Aqueous humor
Aqueous ammonia
Aqueous humor
Occlusio pupillae definition
What is an aqueous solution
Figure 8.3
Additives used in liquid dosage form
Aldol
Chapter 15 water and aqueous systems
Watery humour
Assume that an aqueous solution of a cation
Chapter 15 water and aqueous systems
Why study thermodynamics
Thermodynamics webquest
What is thermodynamics equilibrium
Thermodynamics property tables
Entropy equation
Throttling device
Energy balance equation thermodynamics open system
Thermodynamics deals with
Tds equation
Thermodynamic probability
Oth law of thermodynamics
Newton's third law of thermodynamics
Third law of thermodynamics
Engineering thermodynamics
First law of thermodynamics
1th law of thermodynamics
Residual properties in thermodynamics
Laws of thermodynamics simple
Statistical thermodynamics in chemistry
Maxwell relations
Reversible process
Change in entropy formula
Thermodynamic formula sheet
Thermodynamic equilibrium constant
Coefficient of performance of refrigerator
What is entropy in thermodynamics
Pure substance thermodynamics
Thermodynamics study guide
Maxwell's thermodynamic relations
State second law of thermodynamics
First law of thermodynamics joule's experiment
What is cp in thermodynamics
What is brayton cycle in thermodynamics
Reschual
Chemical engineering thermodynamics 8th solution chapter 3
Thermodynamics for chemical engineering
Internal energy
Gibbs free energy
Raoult's law and dalton's law
Example of adiabatic process
First law of td
In a flow process the work transfer may be of which type
Total entropy
çengel
What is brayton cycle in thermodynamics