Organic Chemistry Sh javanshir Faculty of Chemistry Iran
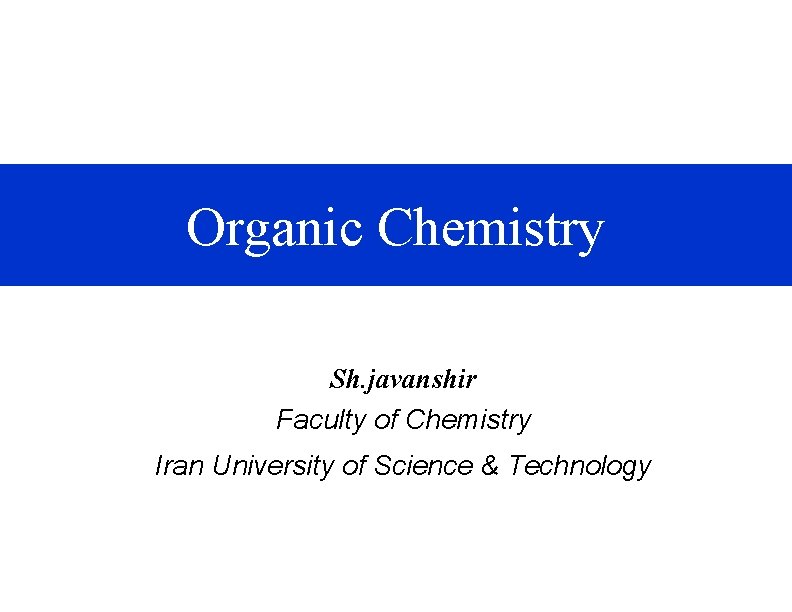
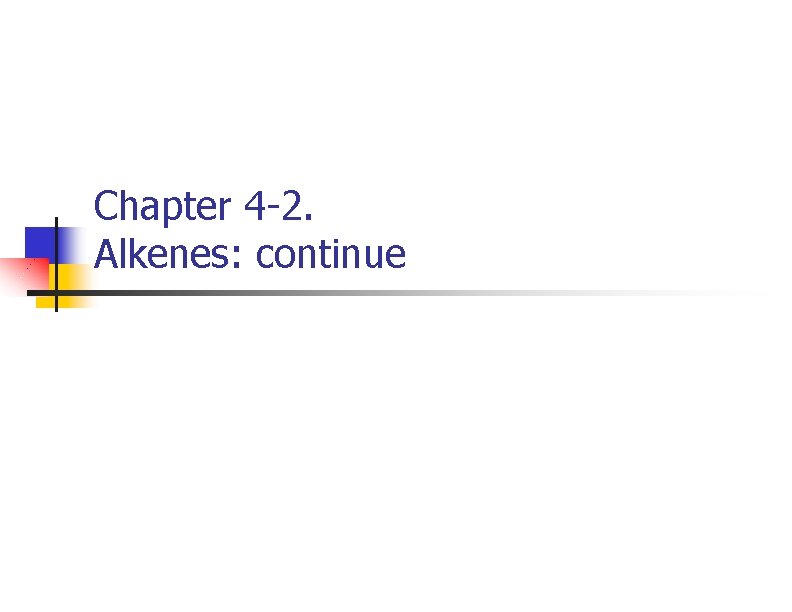
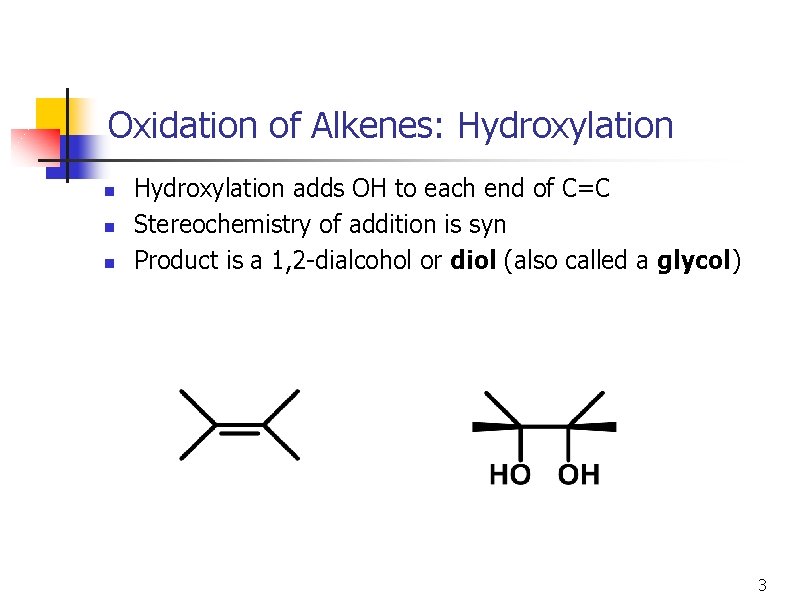
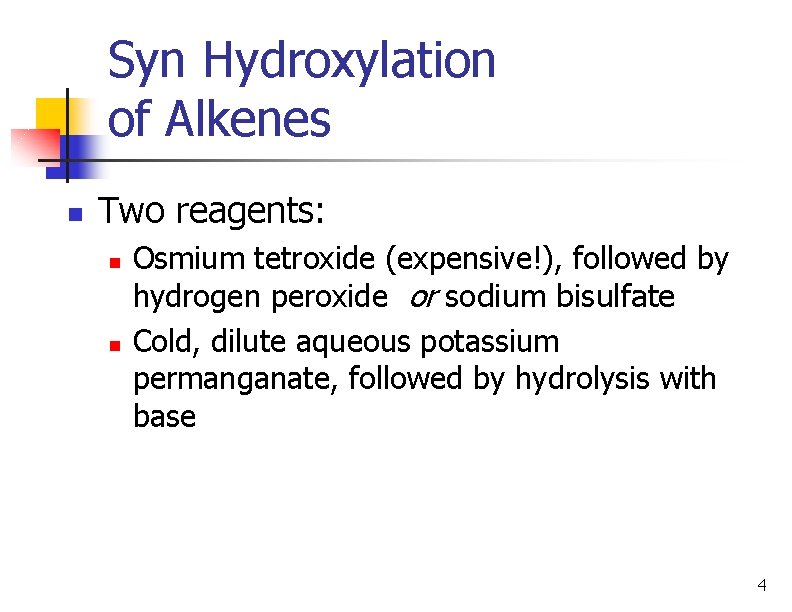
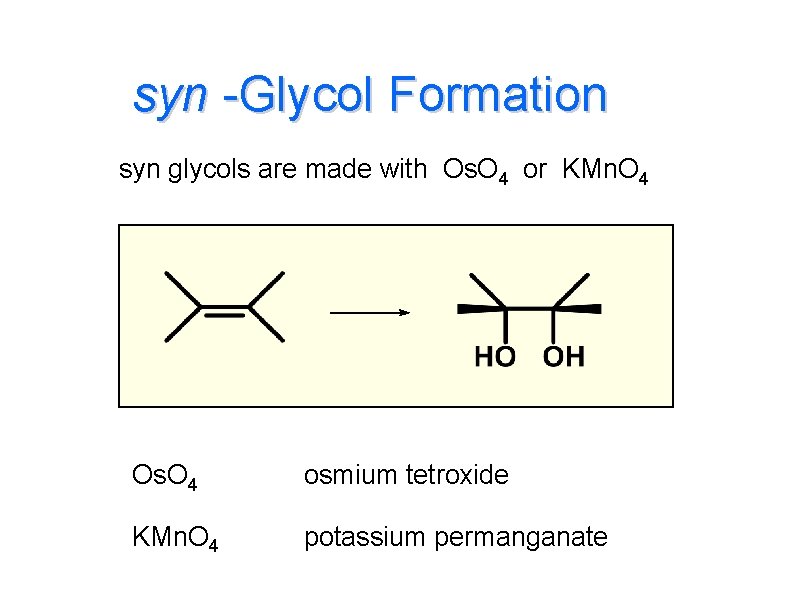
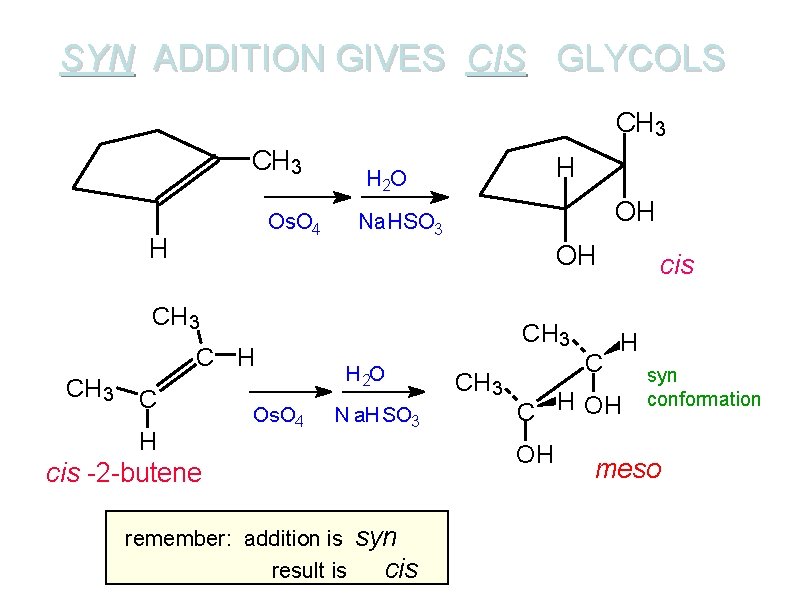
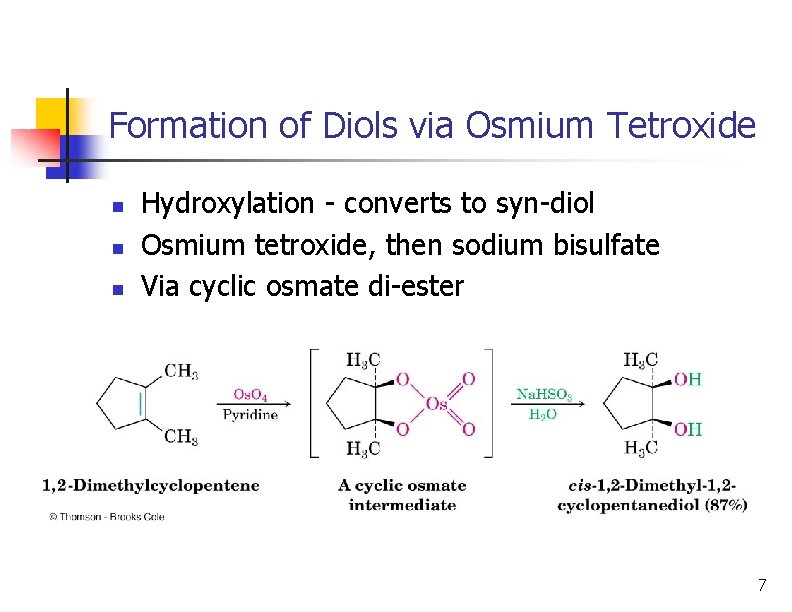
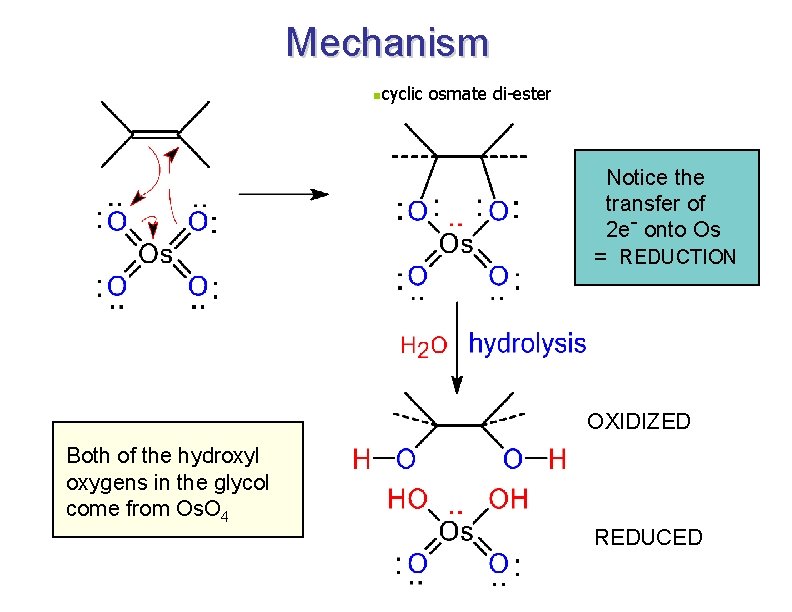
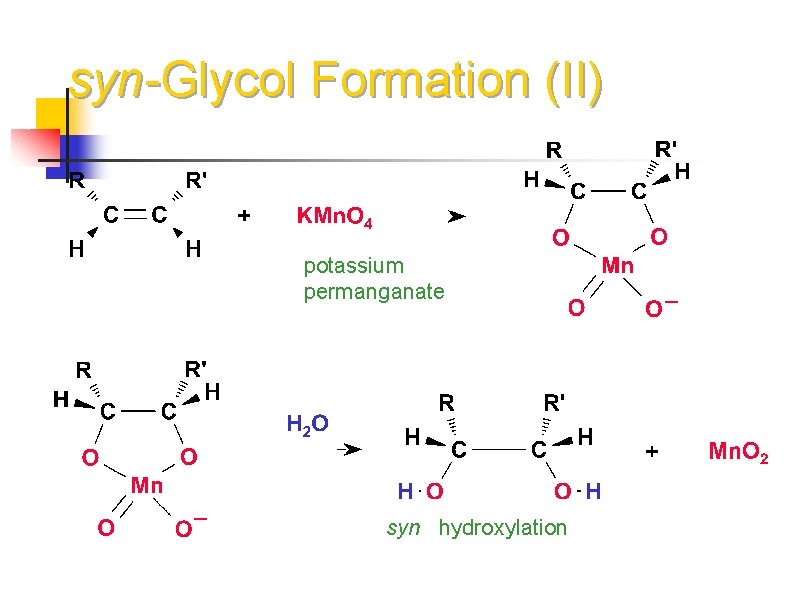
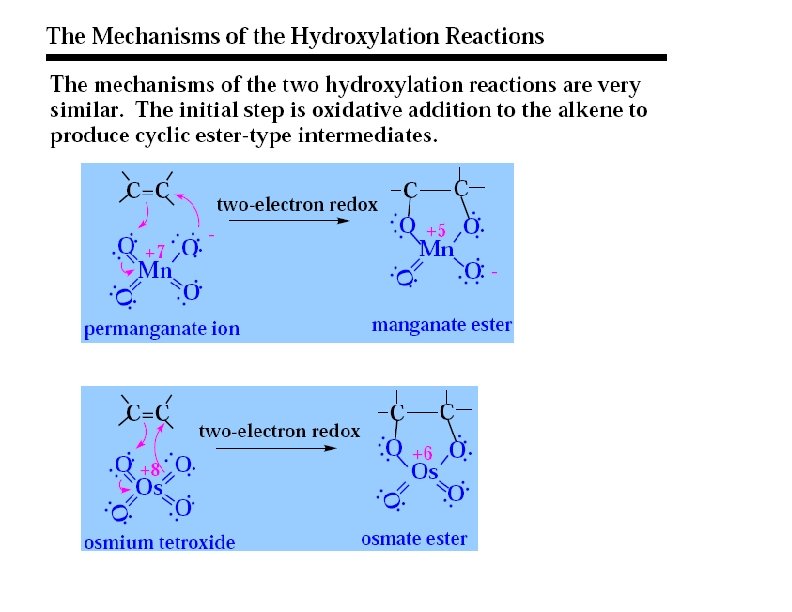
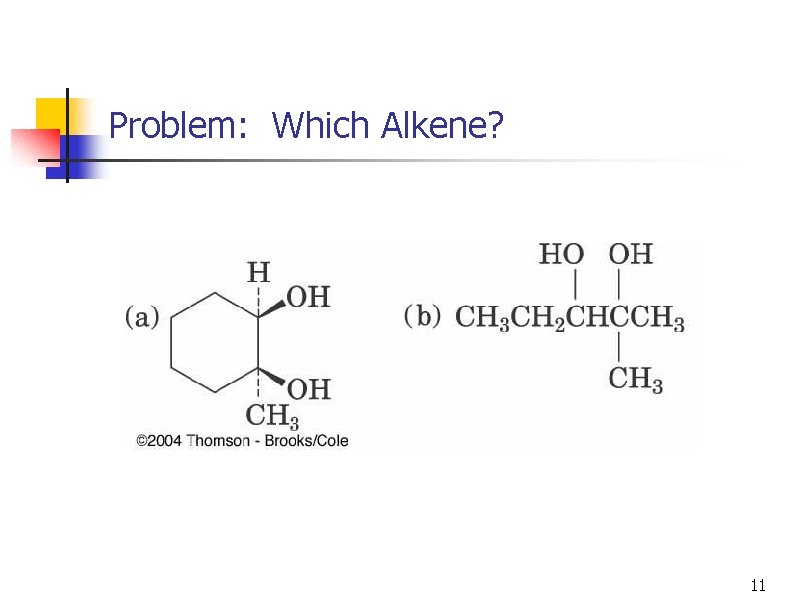
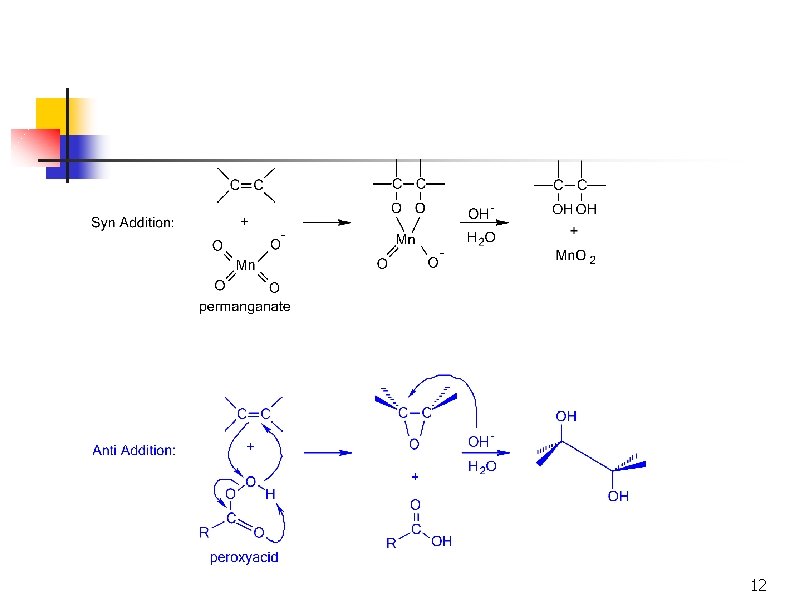
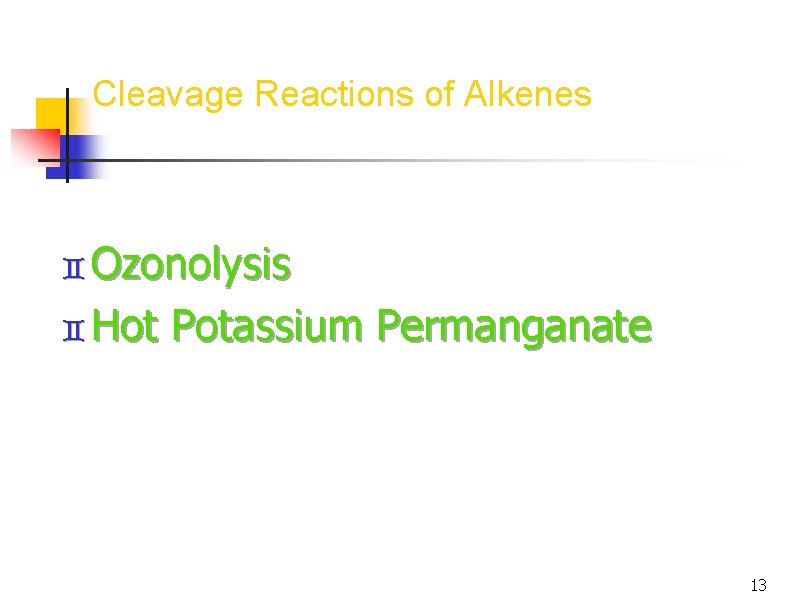
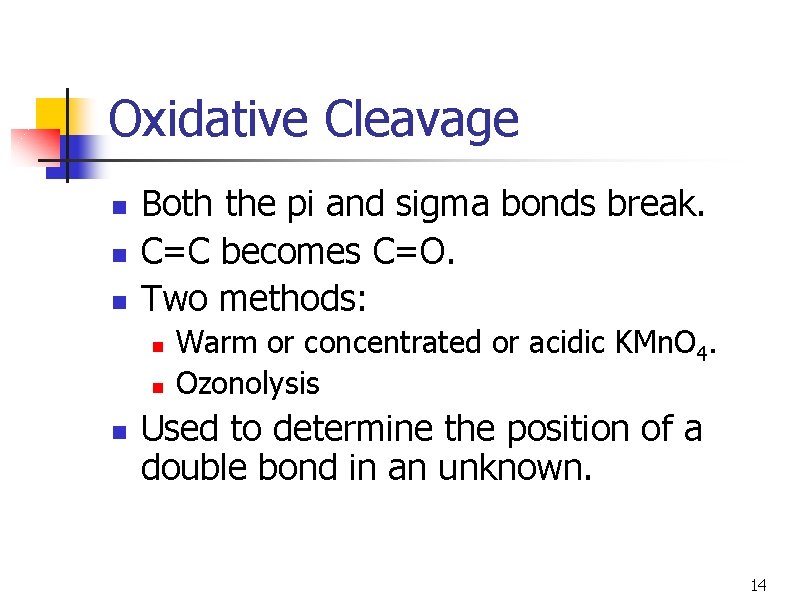
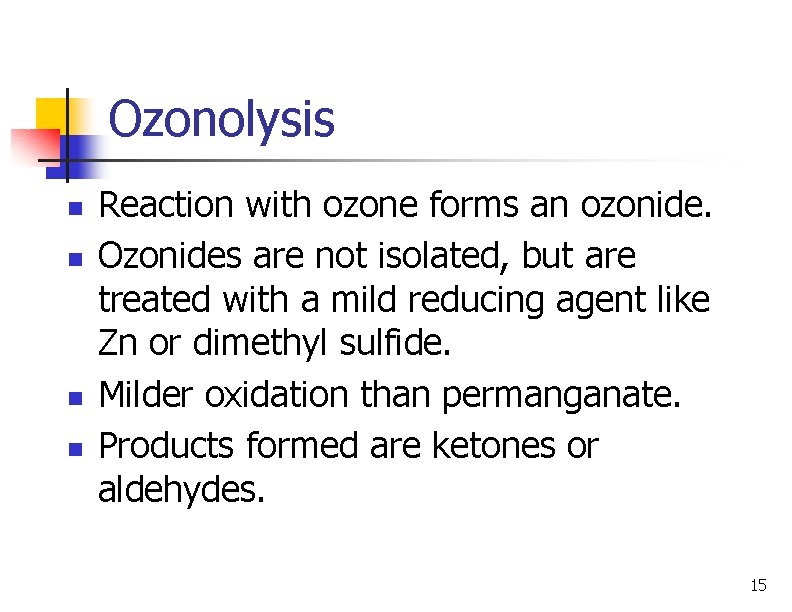
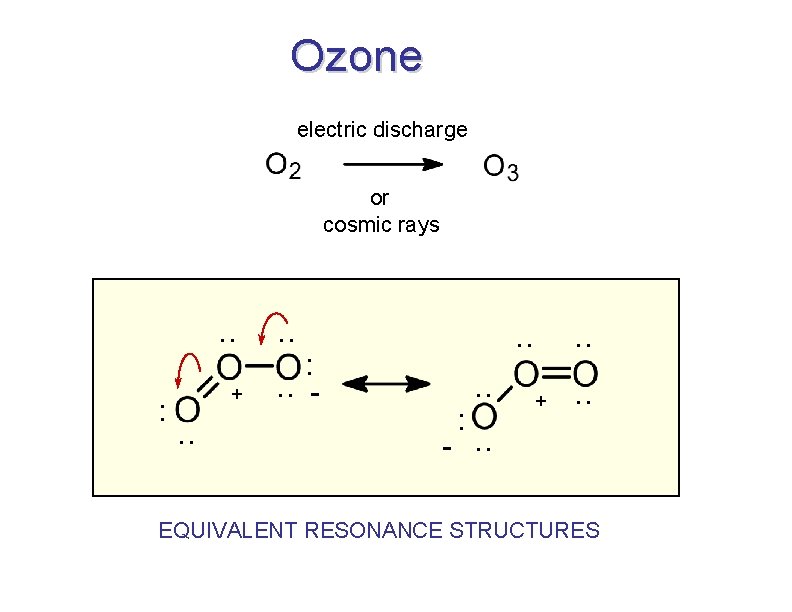
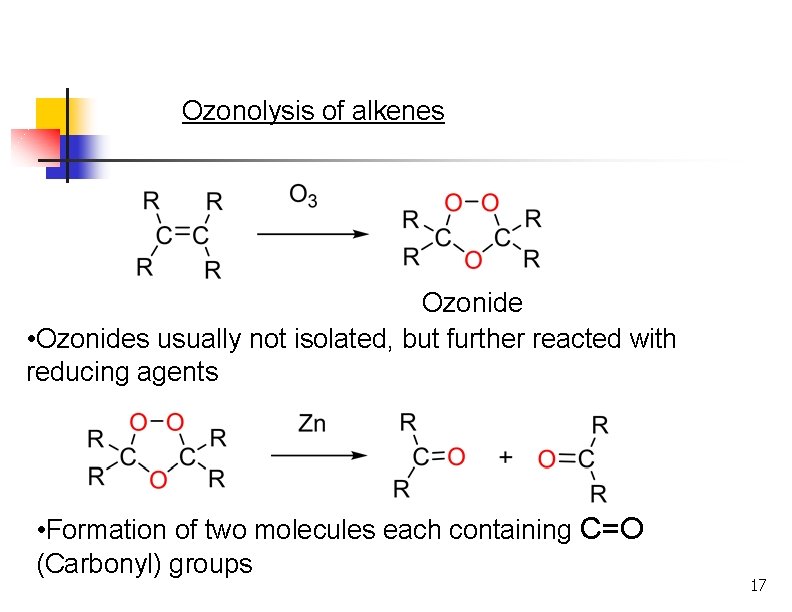
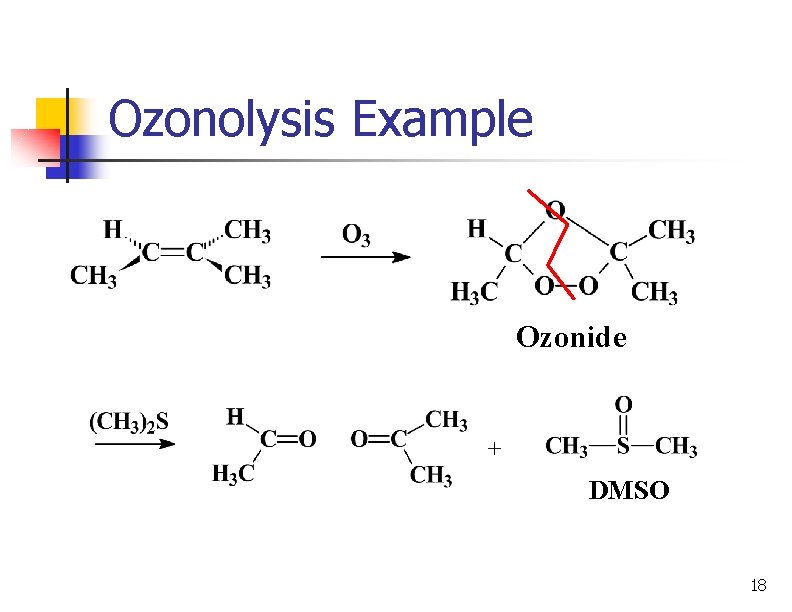
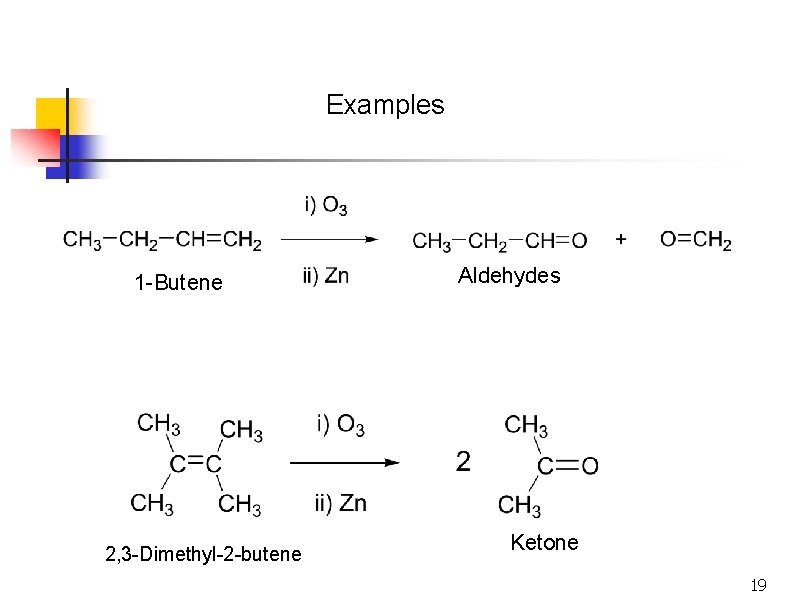
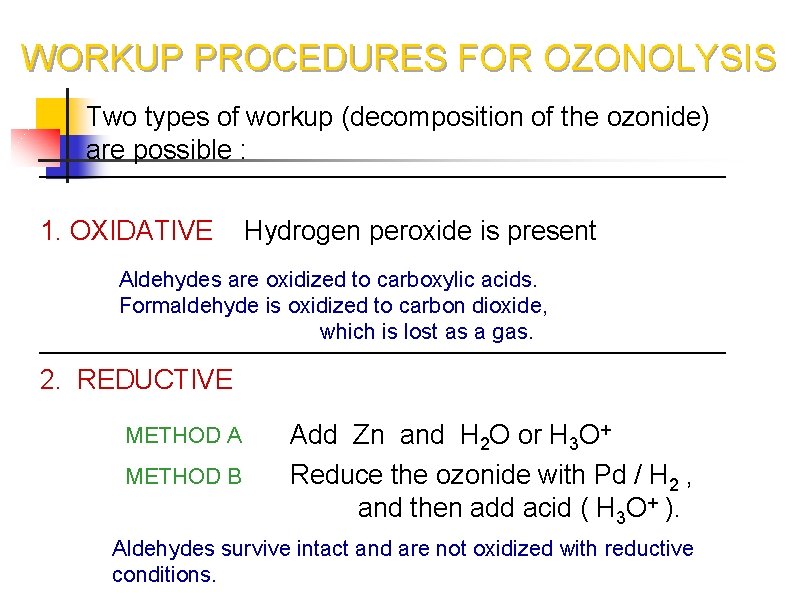
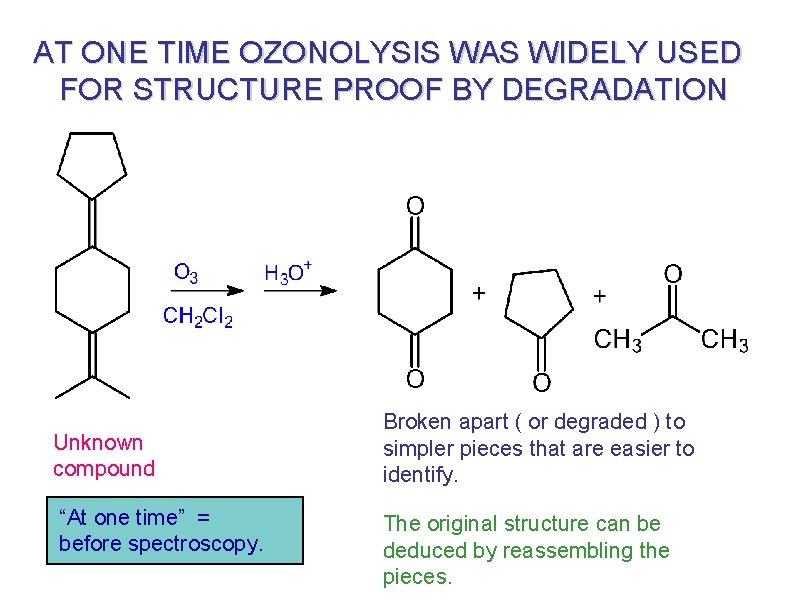
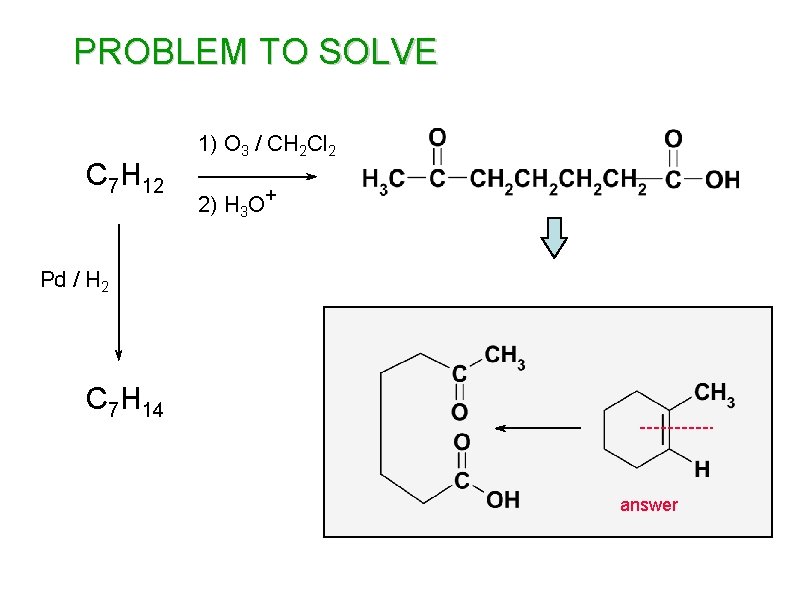
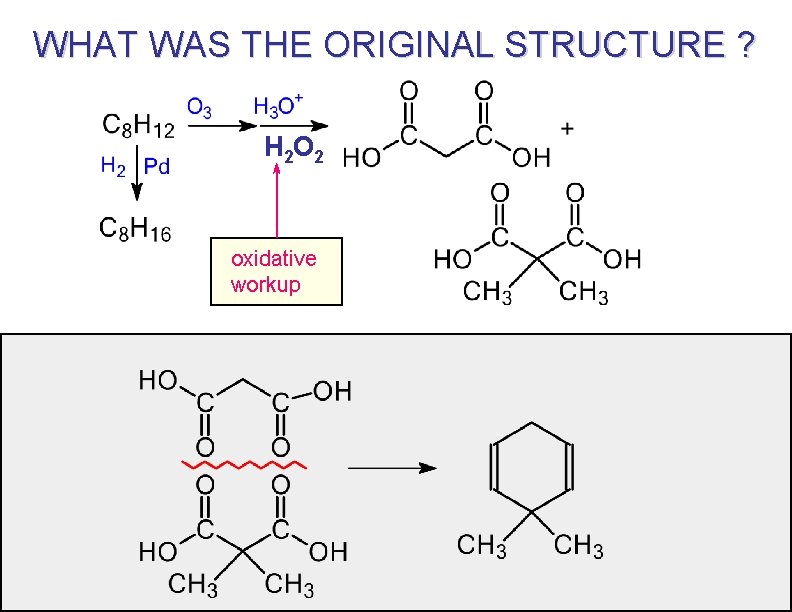
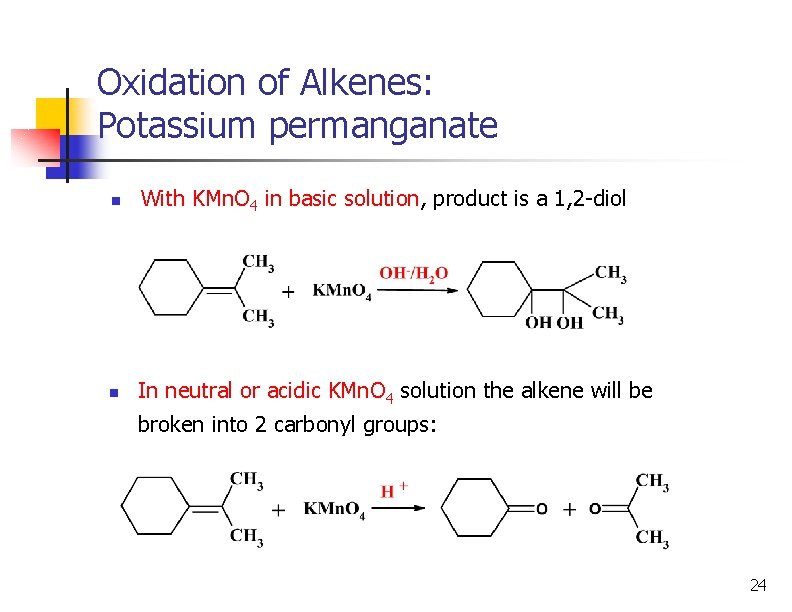
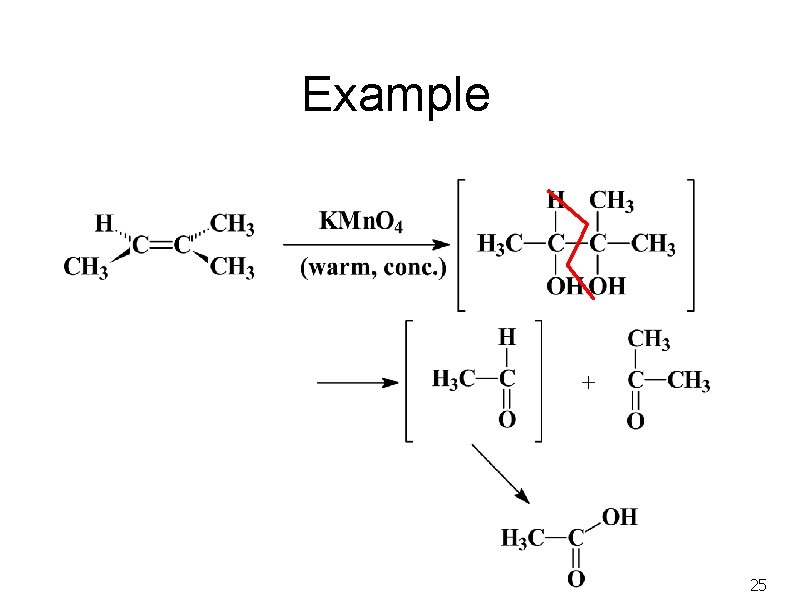
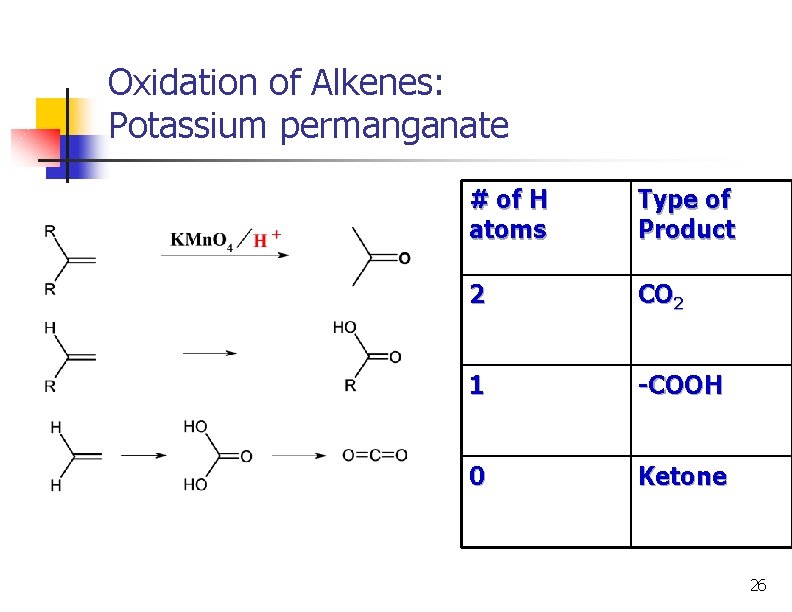
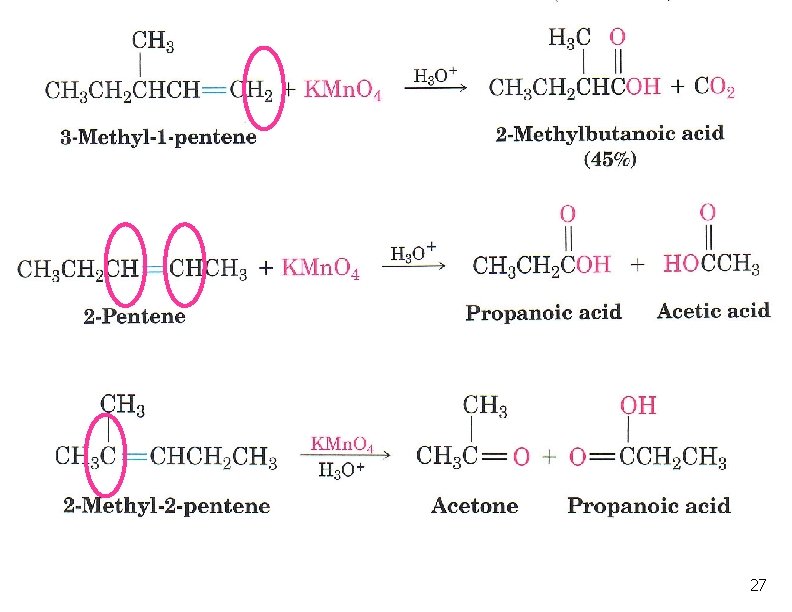
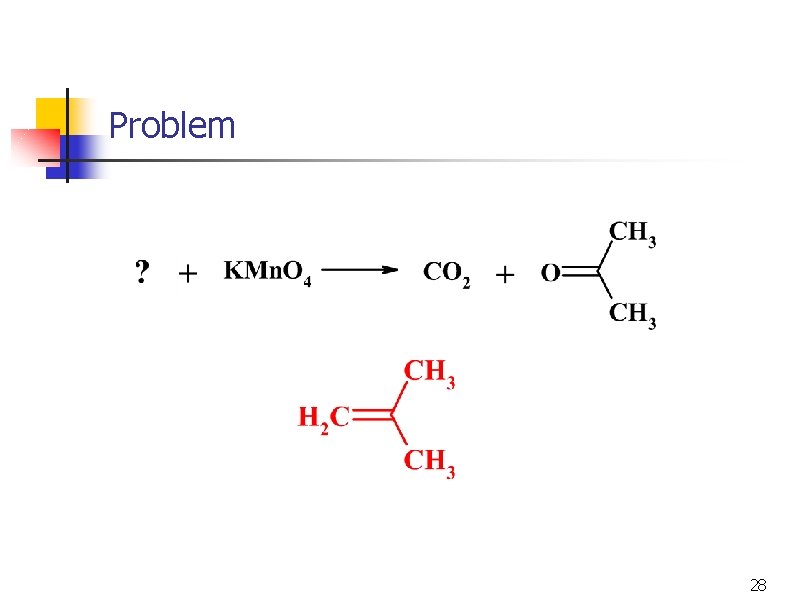
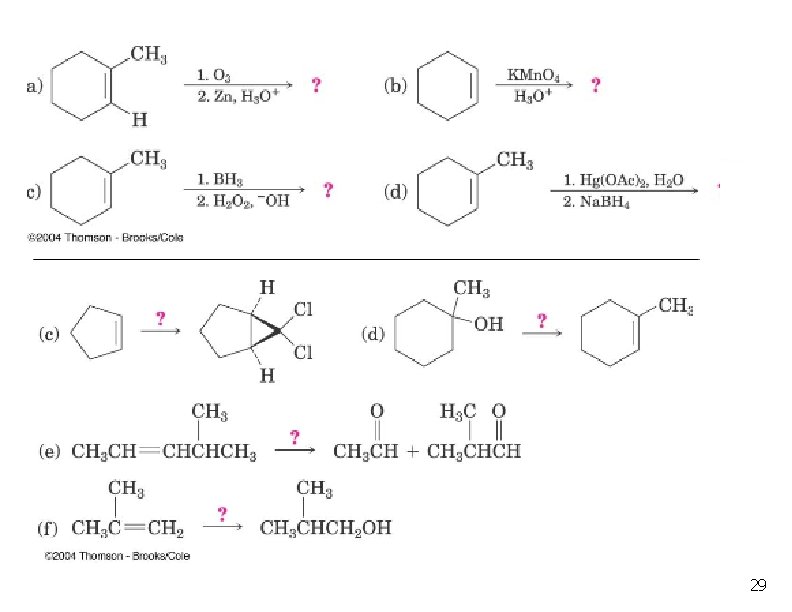
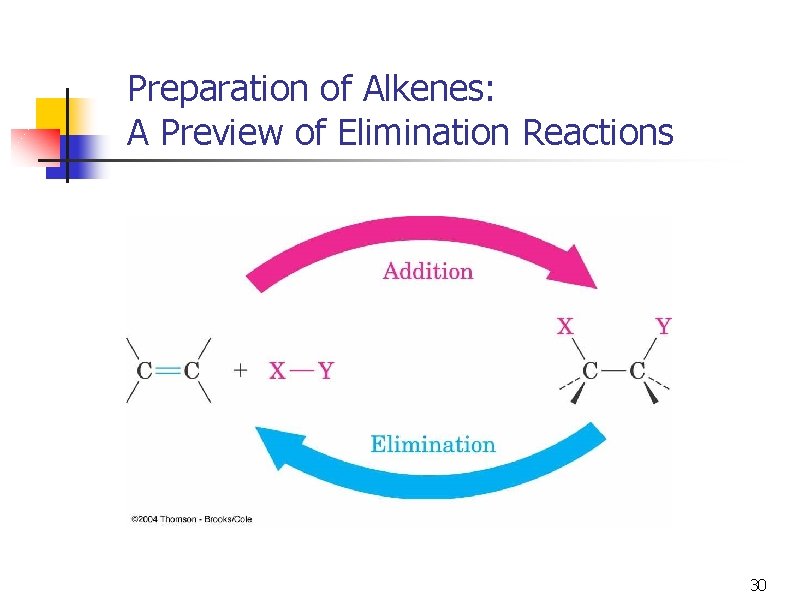
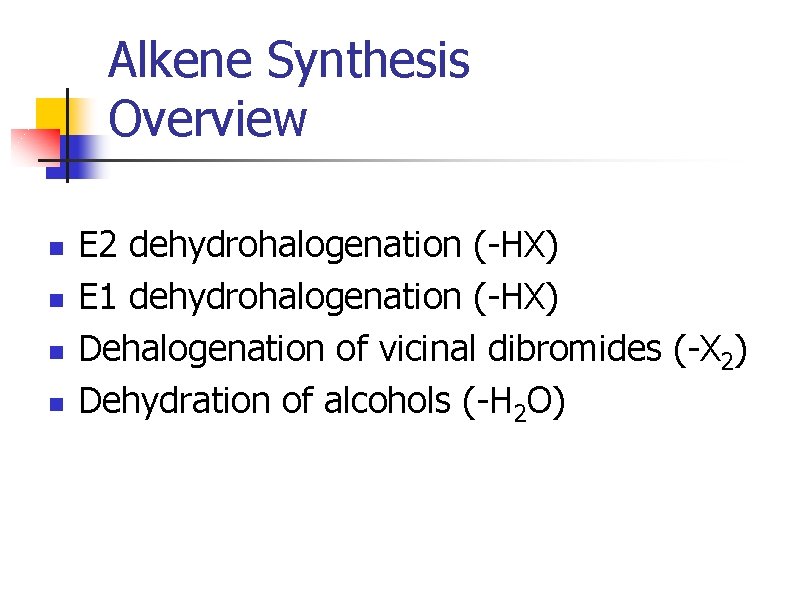
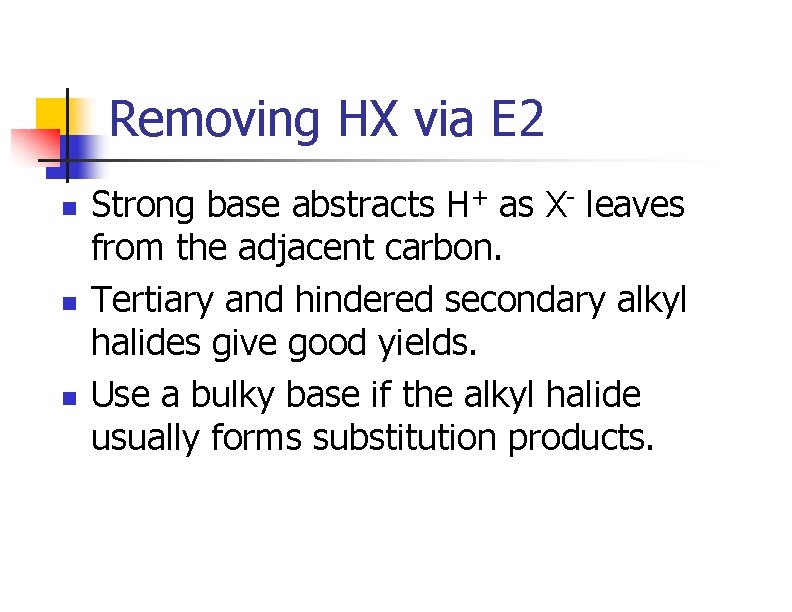
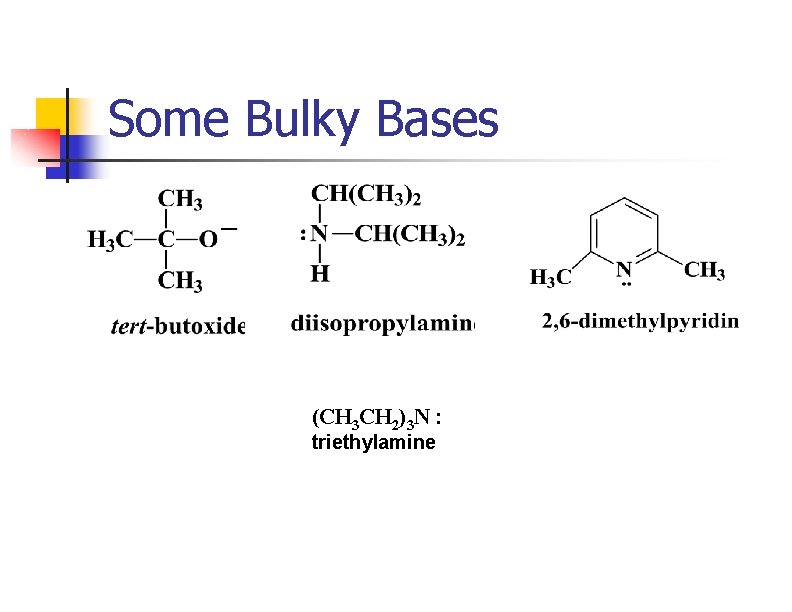
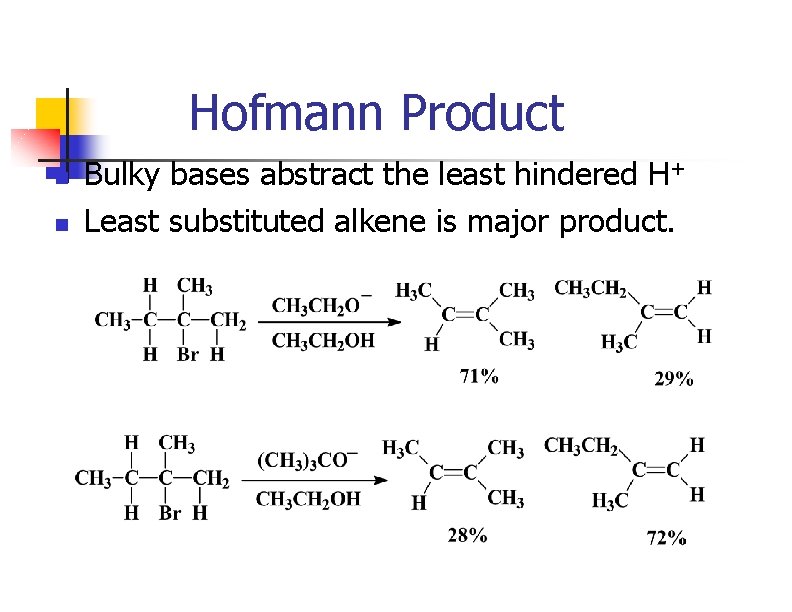
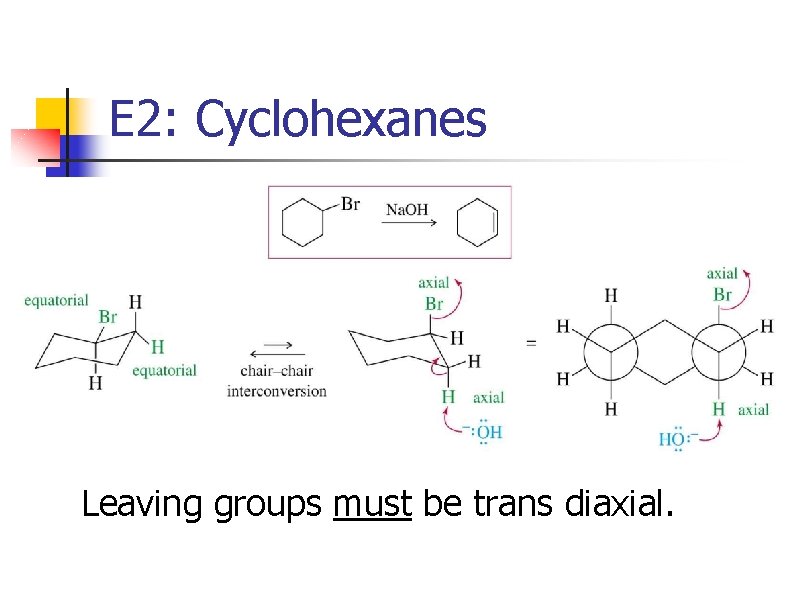
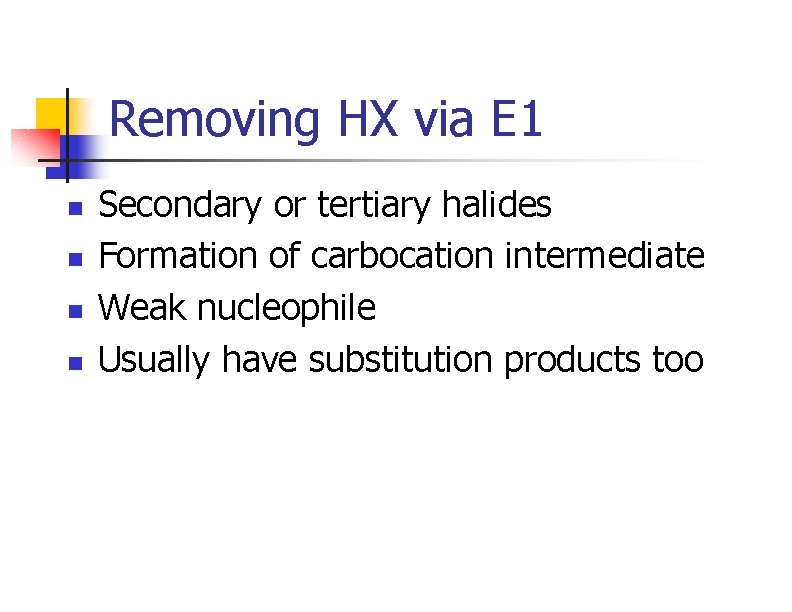
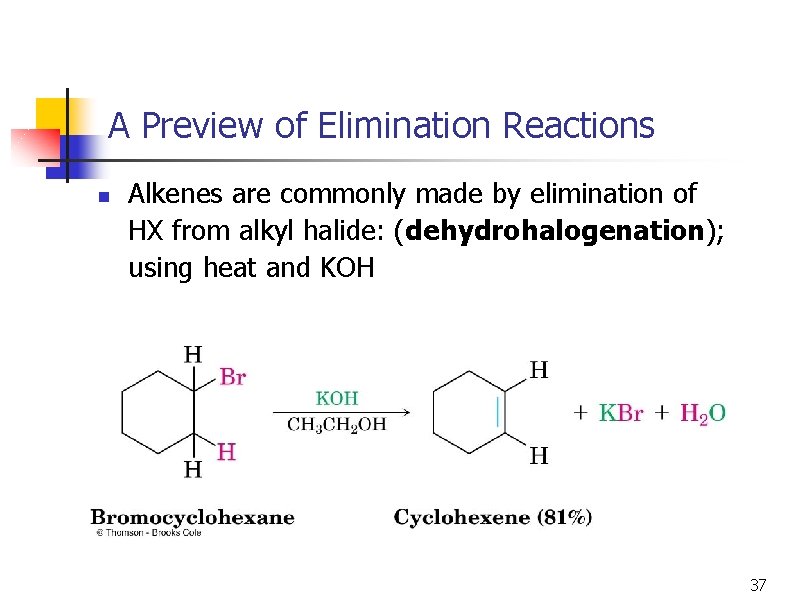
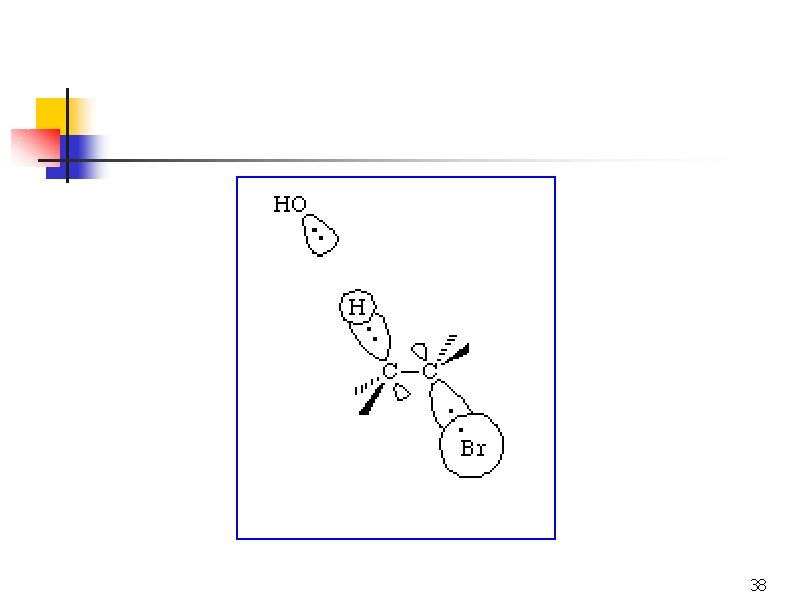
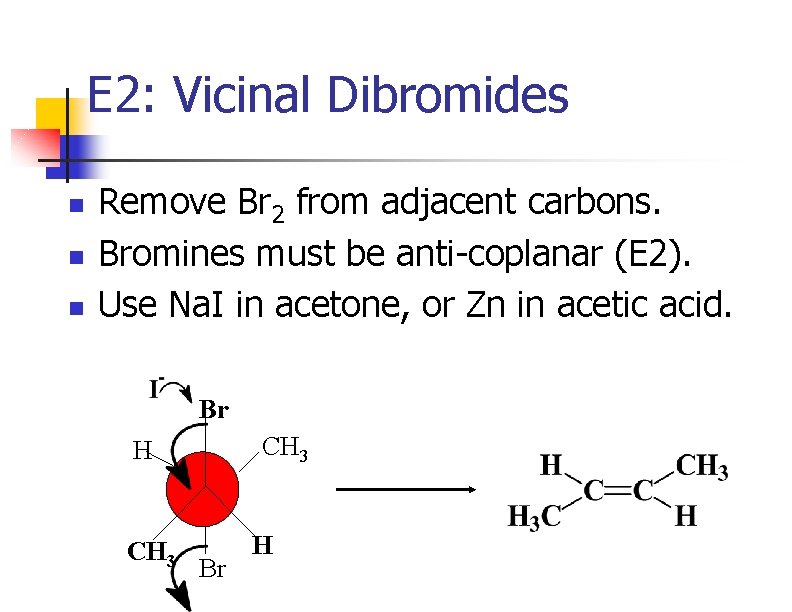
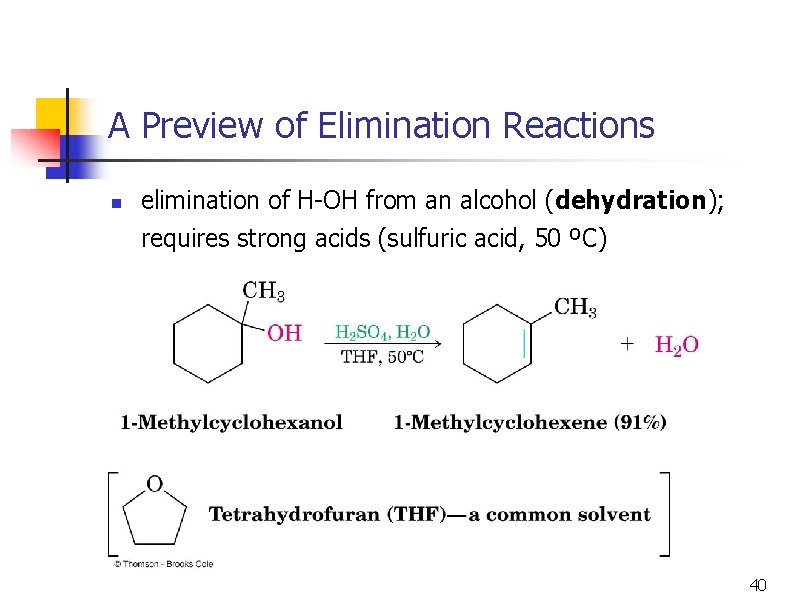
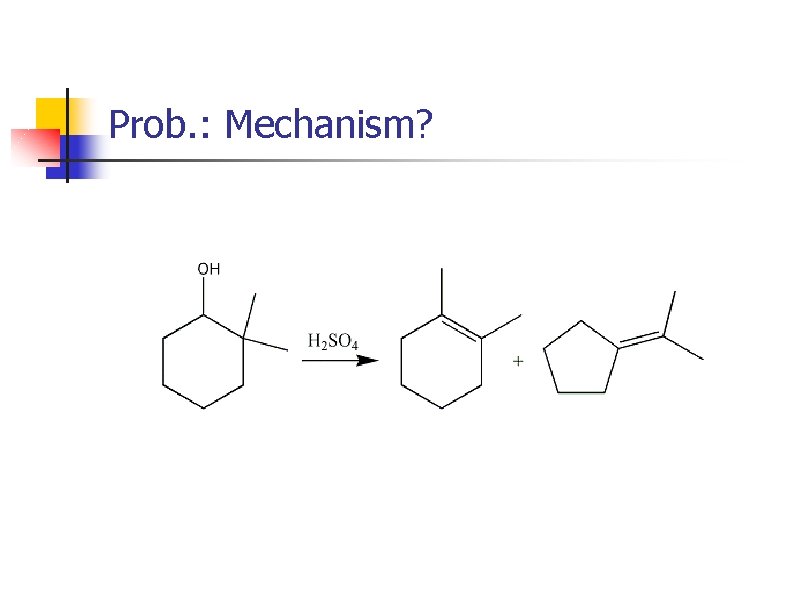
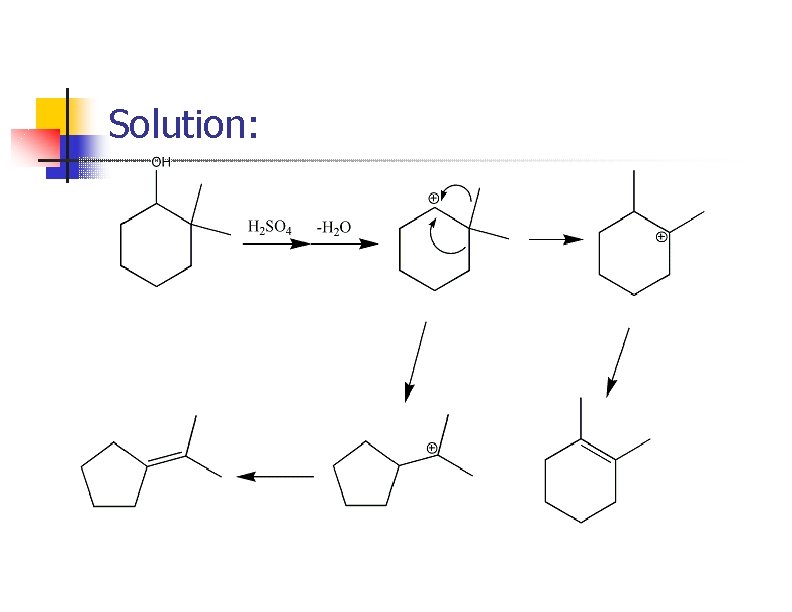
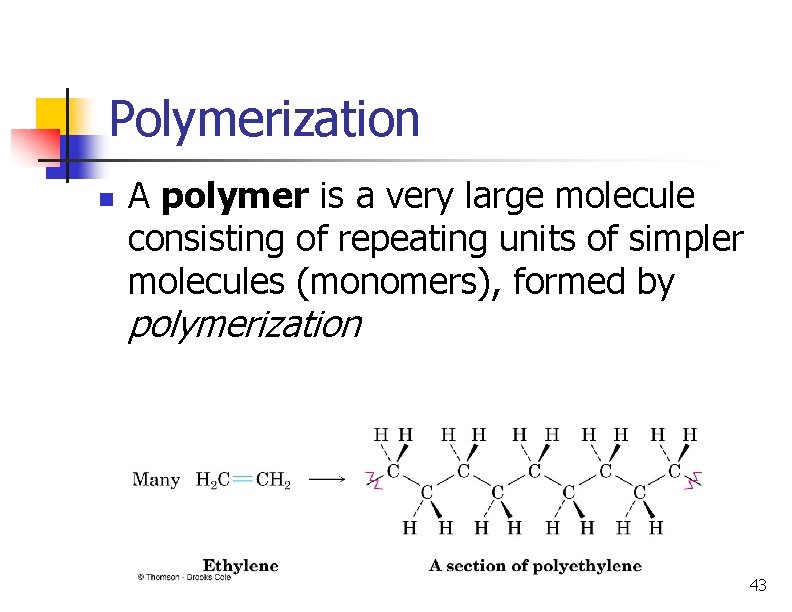
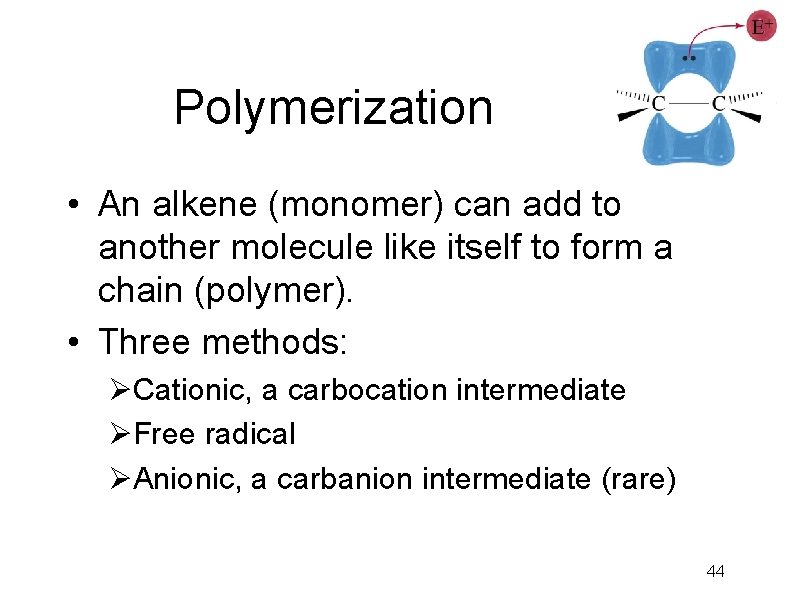
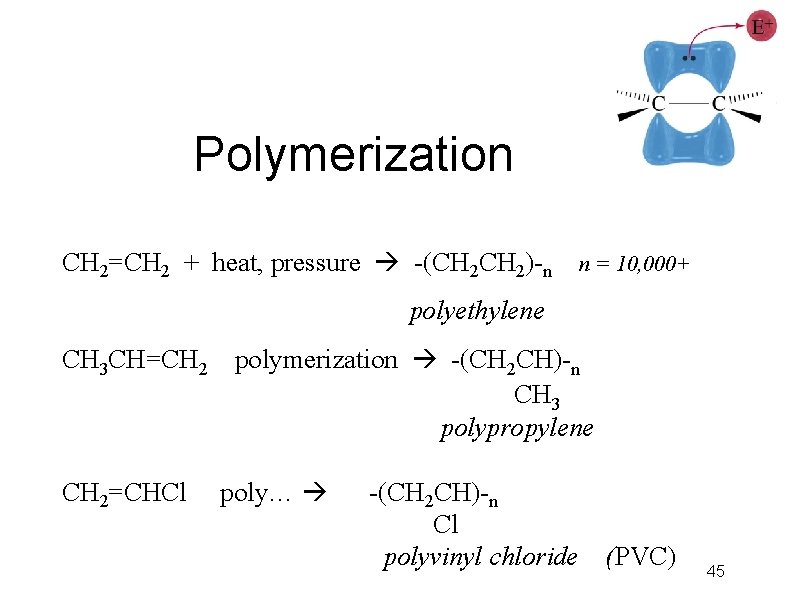
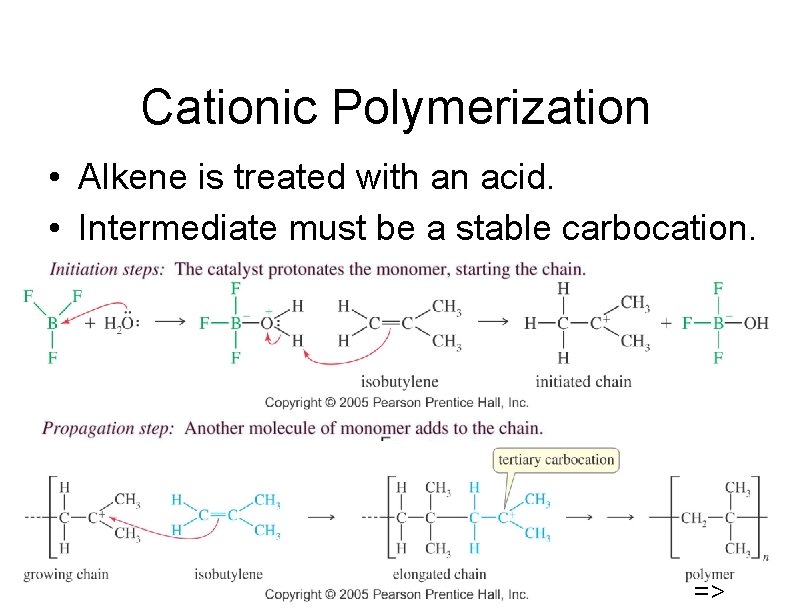
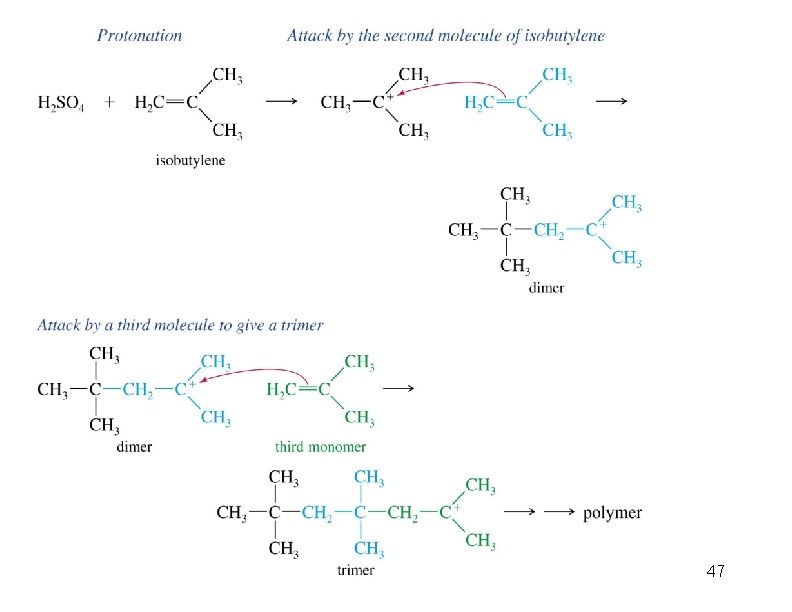
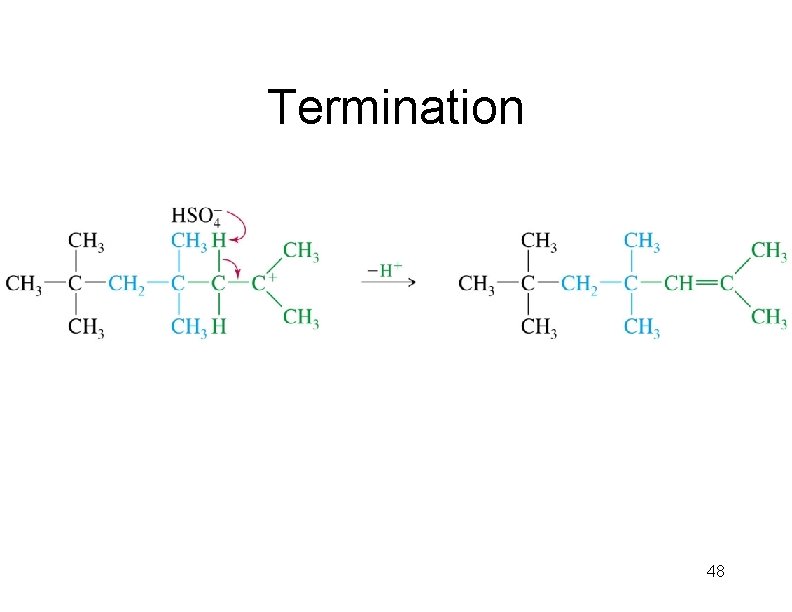
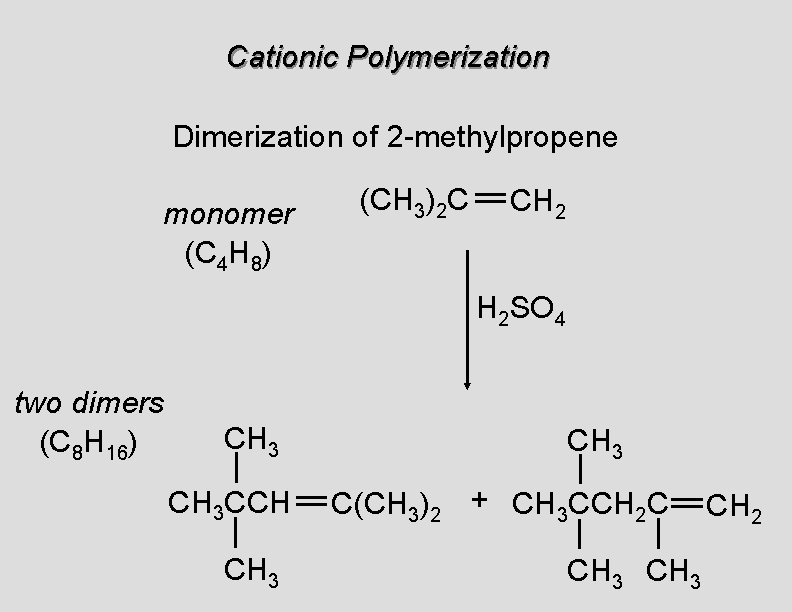
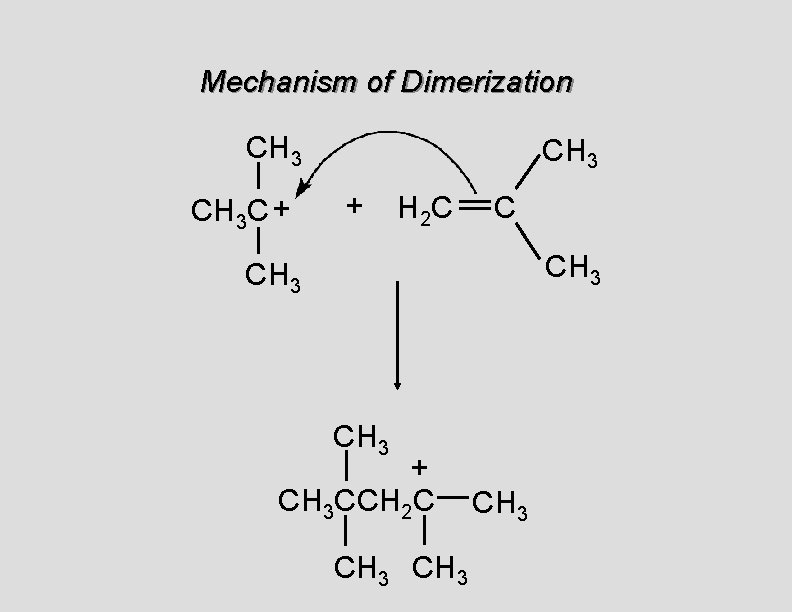
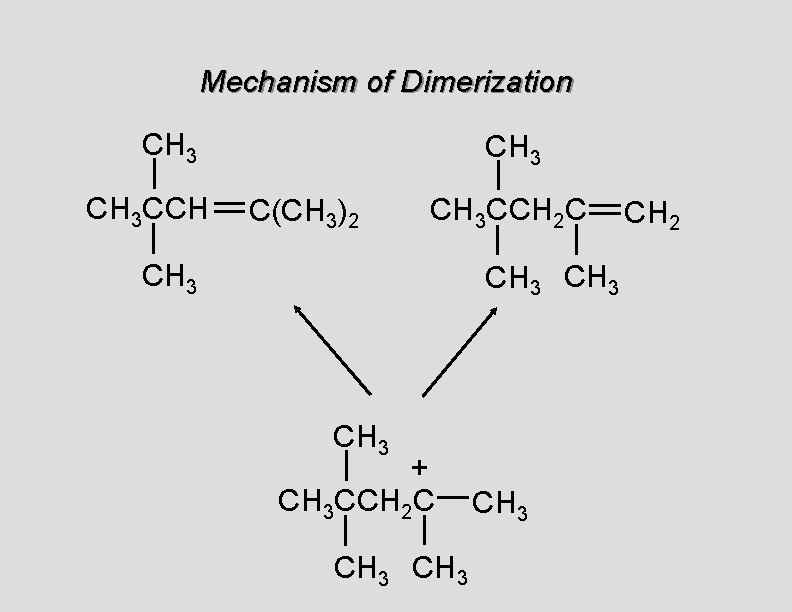
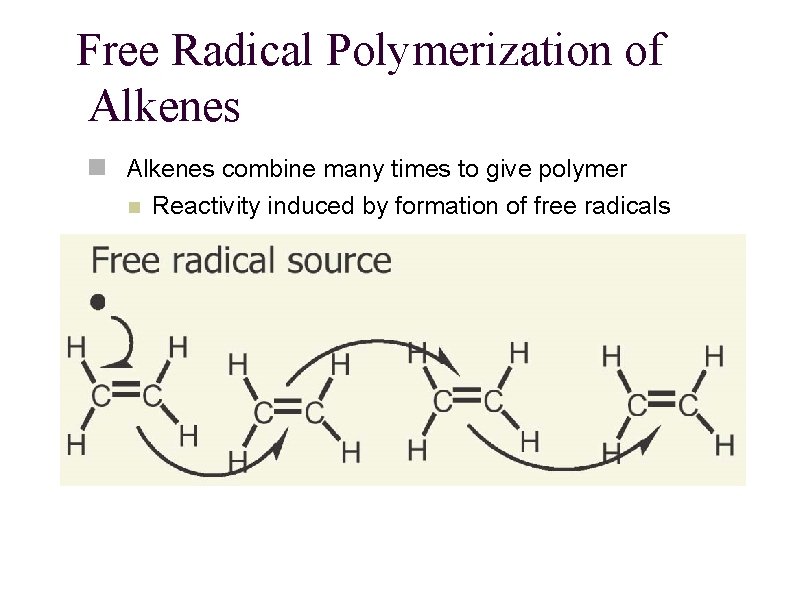
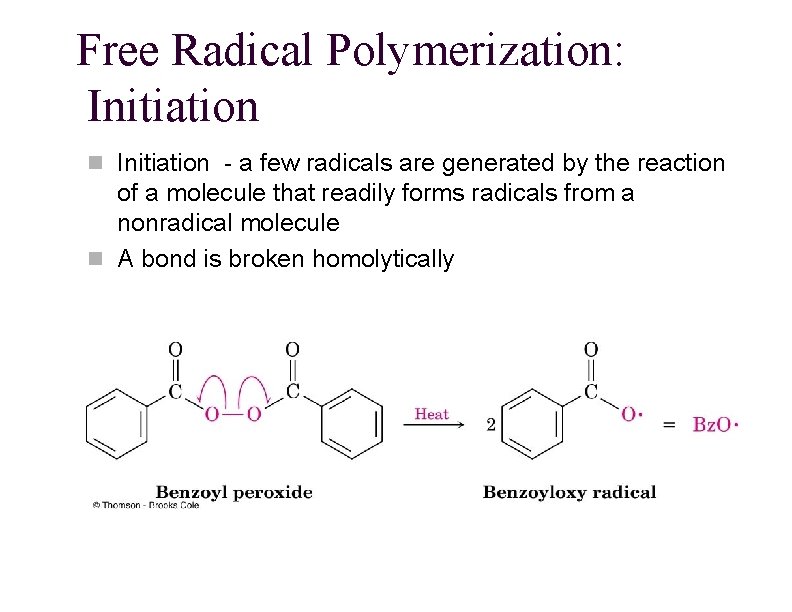
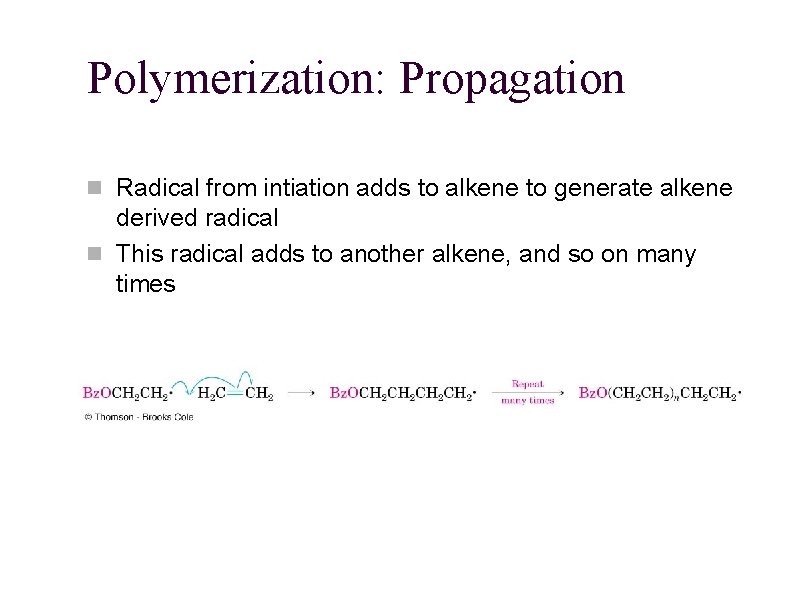
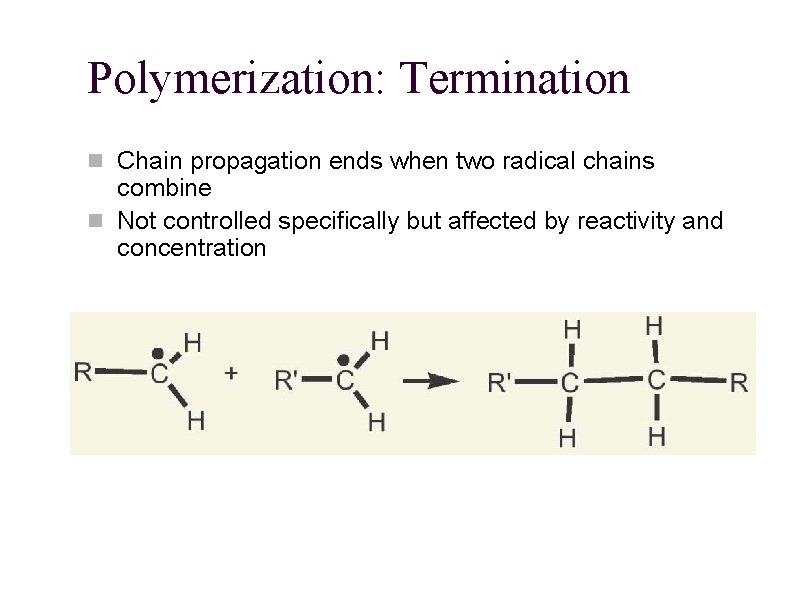
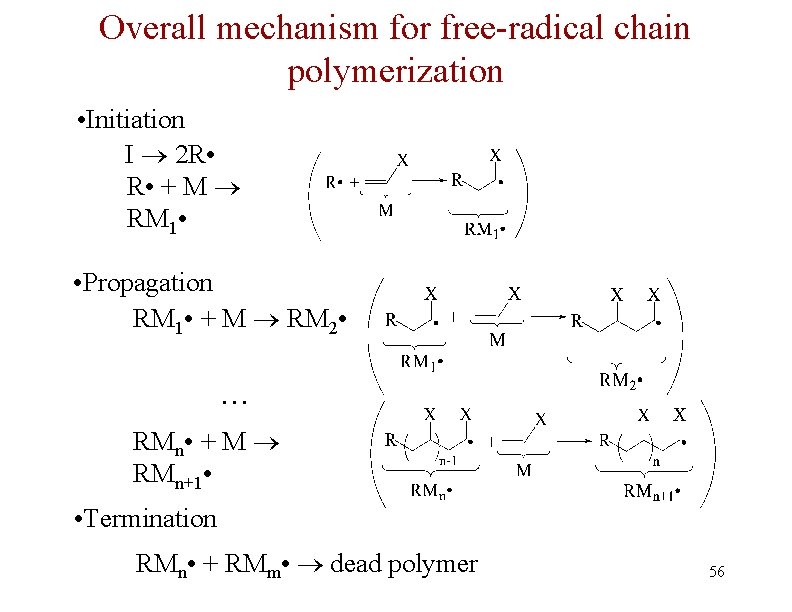
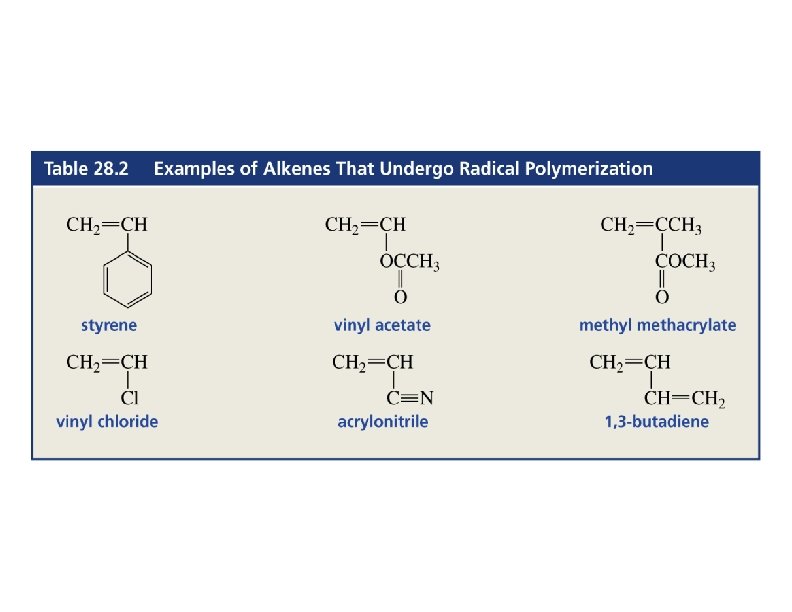
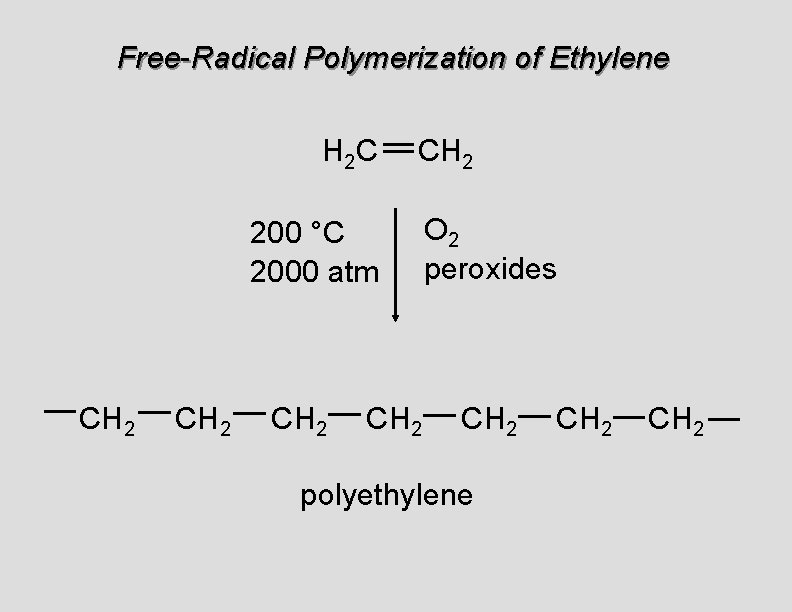
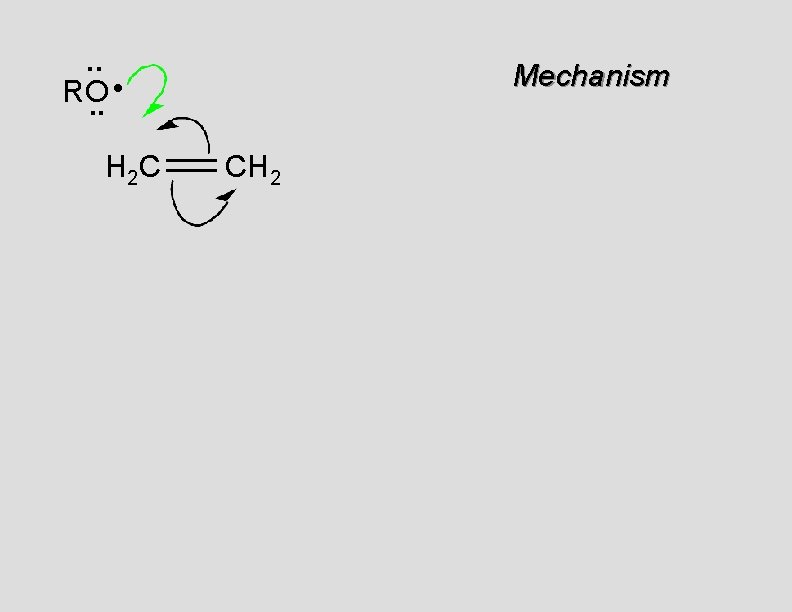
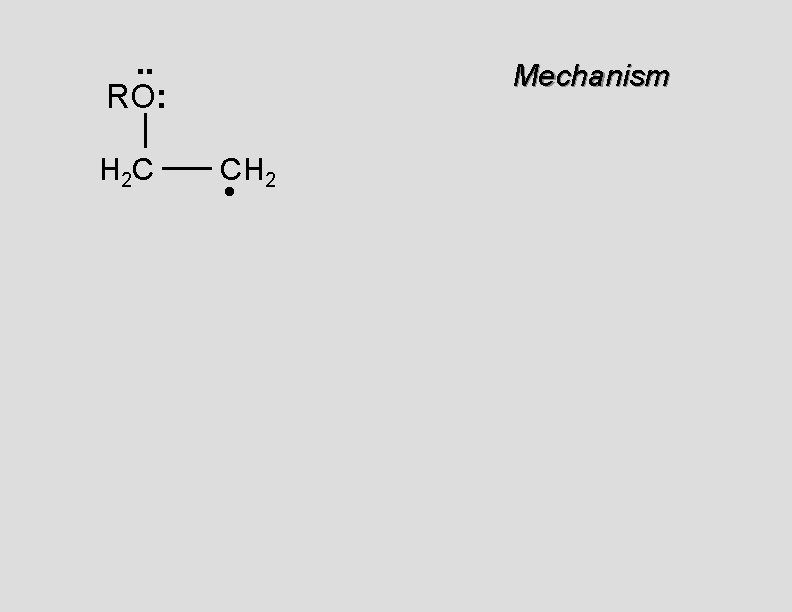
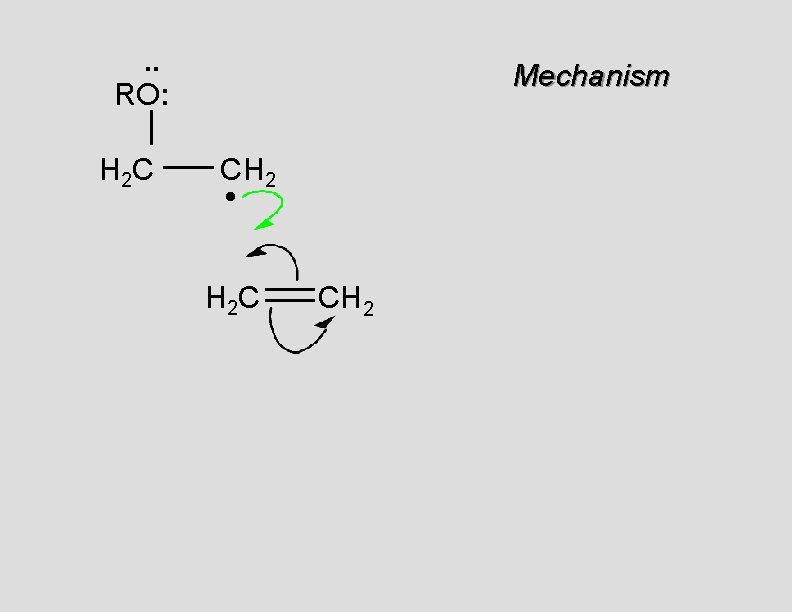
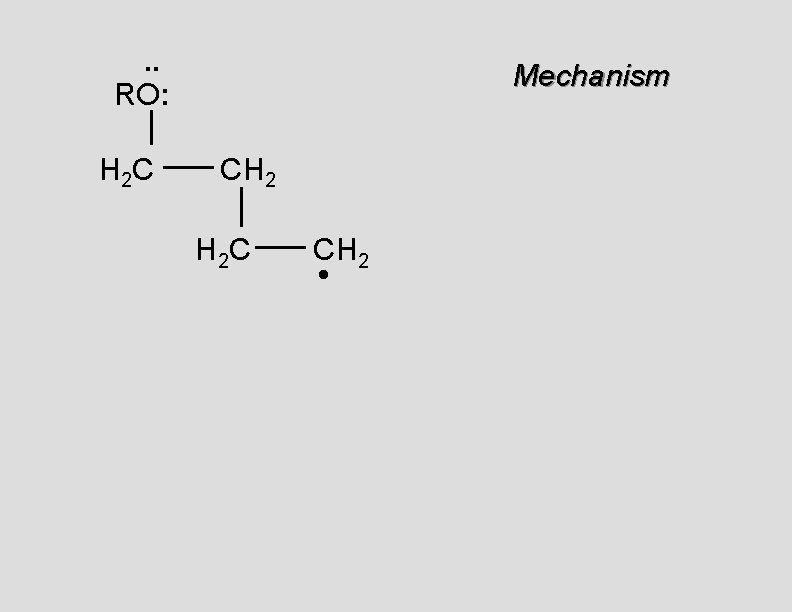
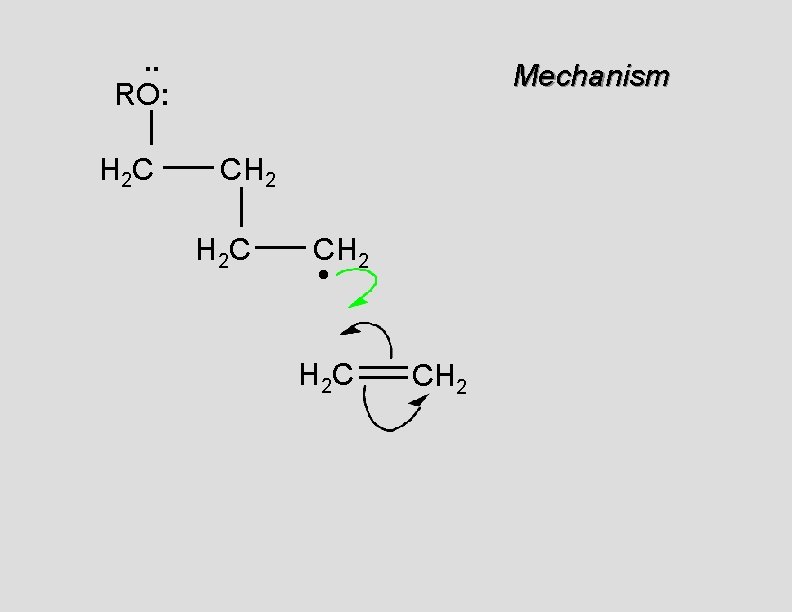
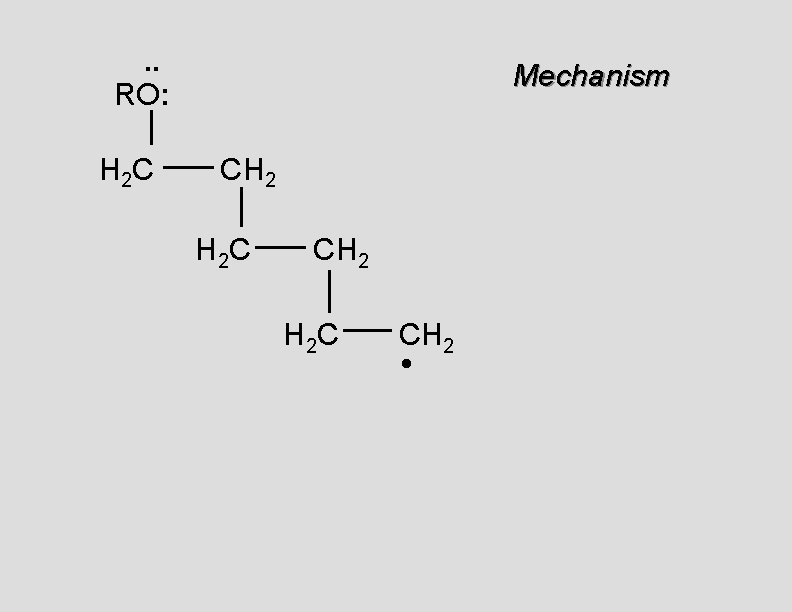
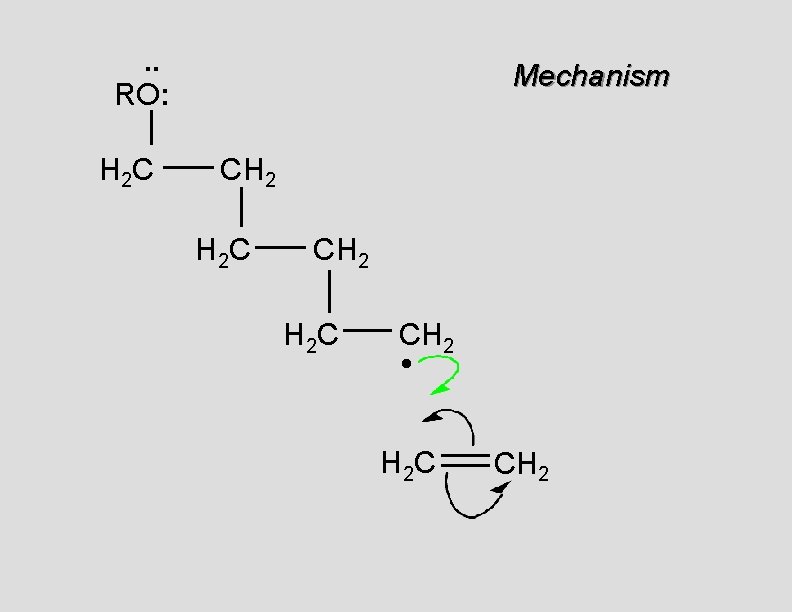
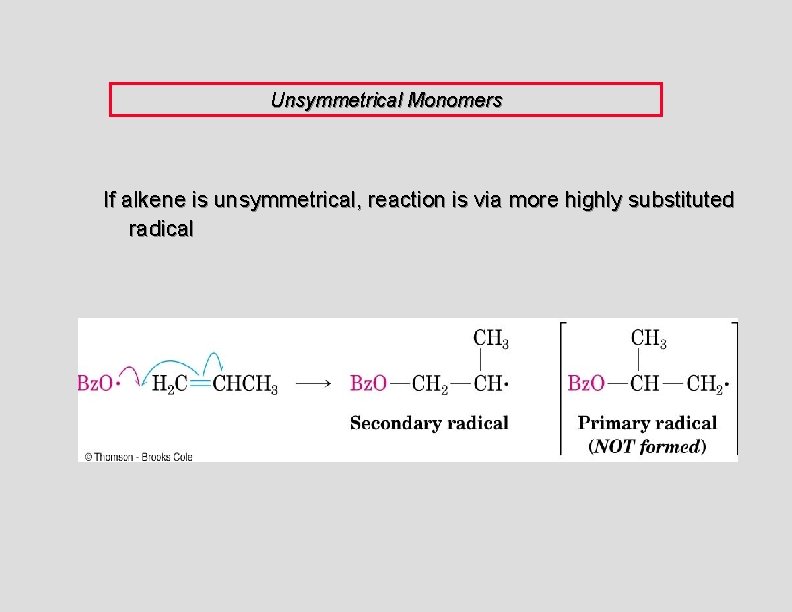
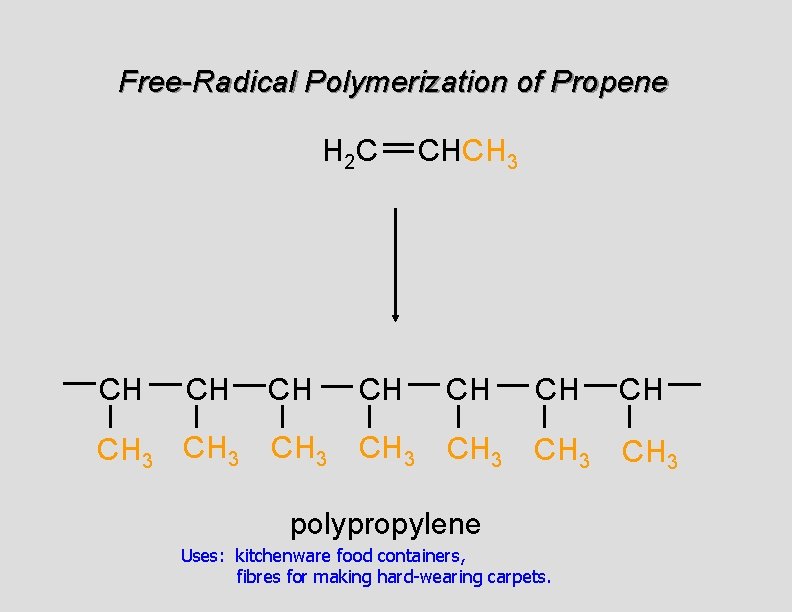
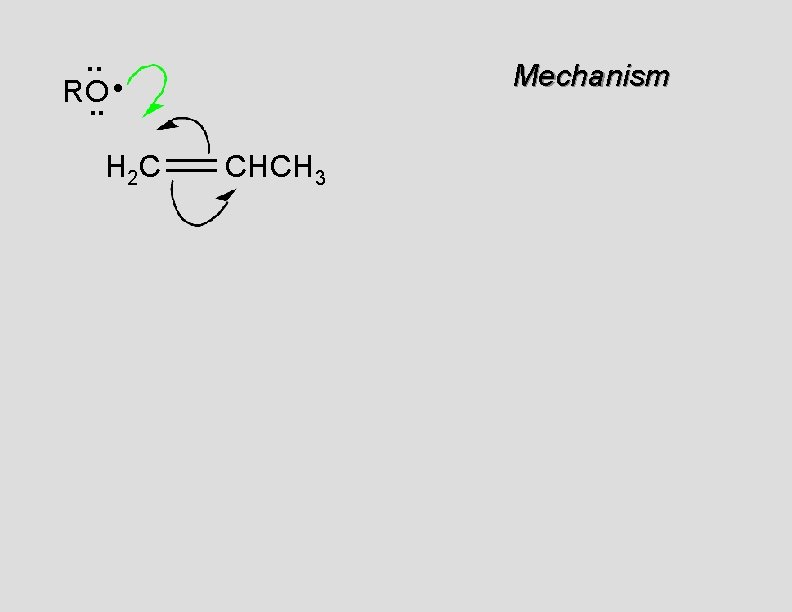
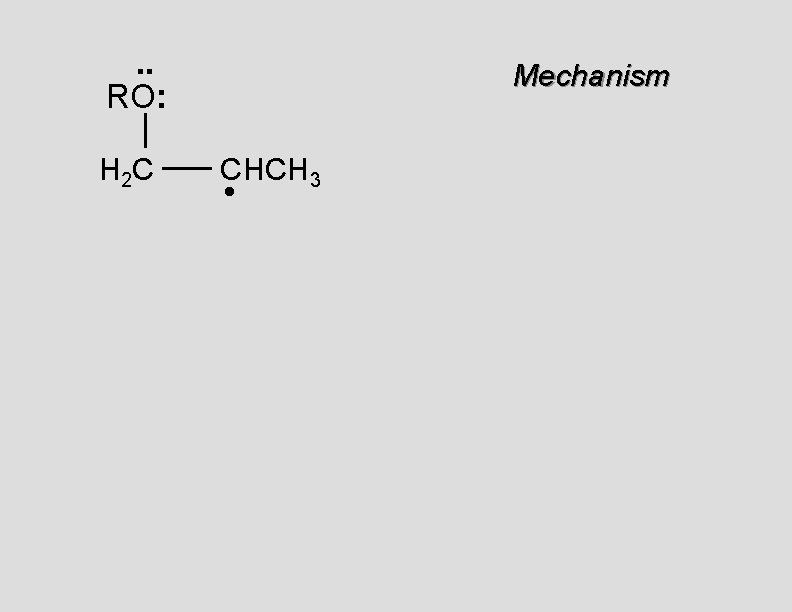
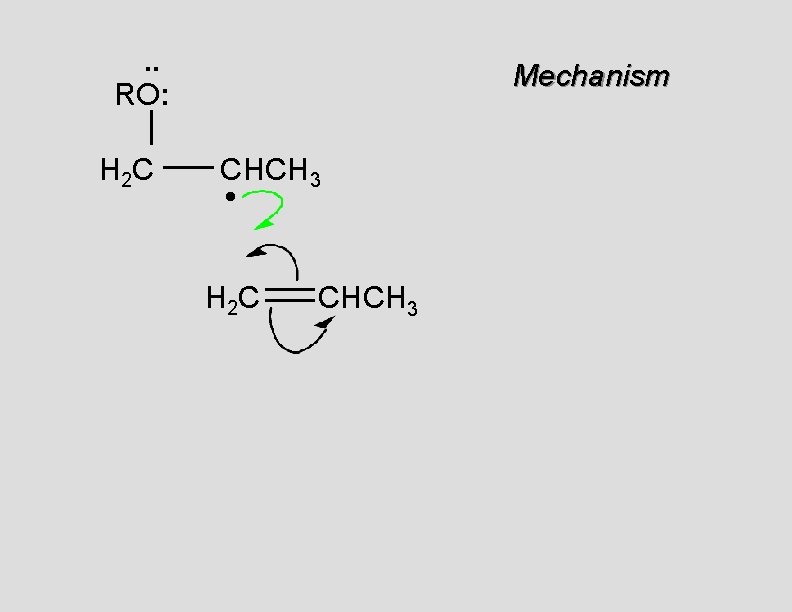
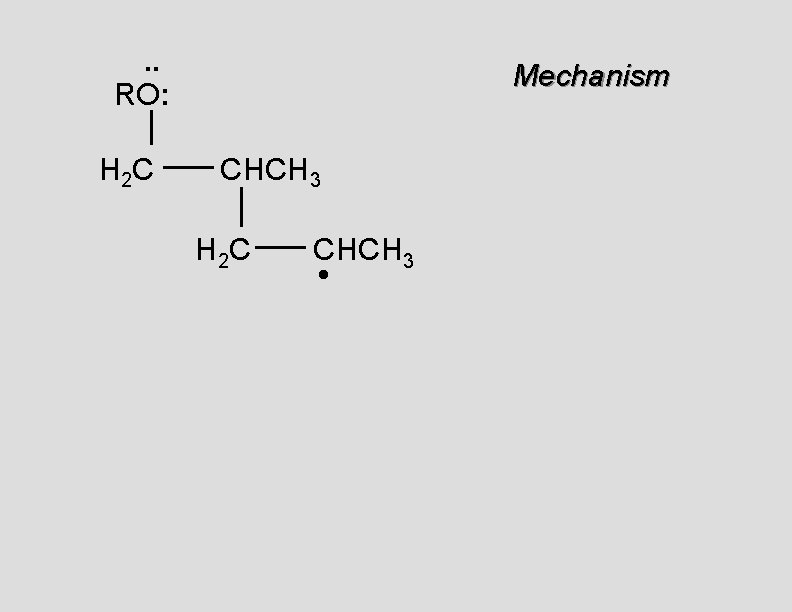
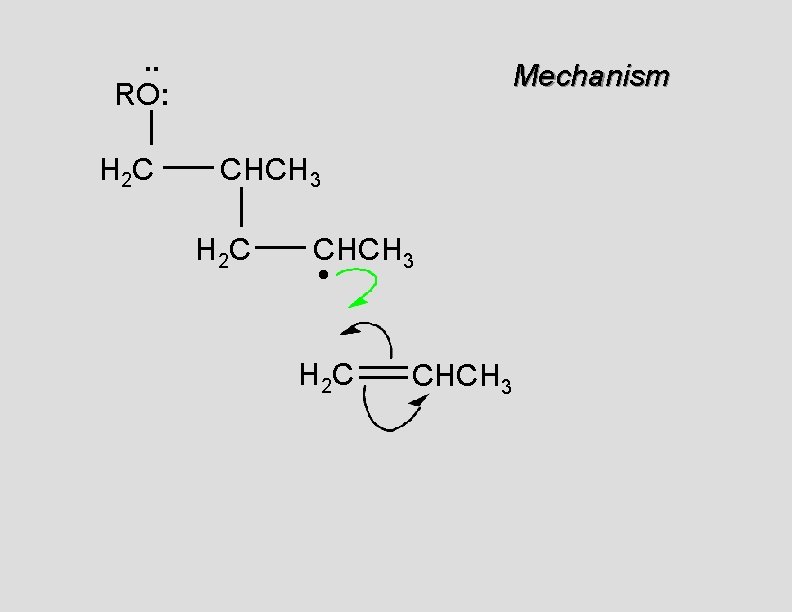
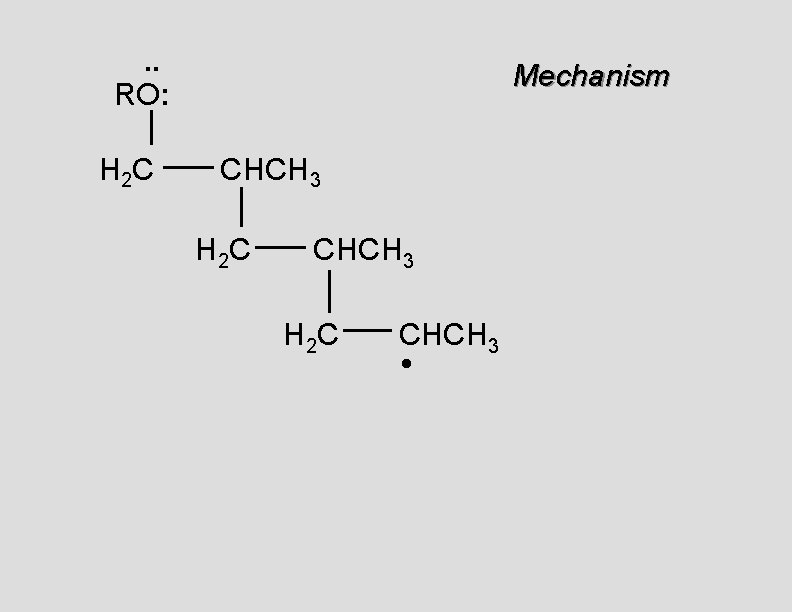
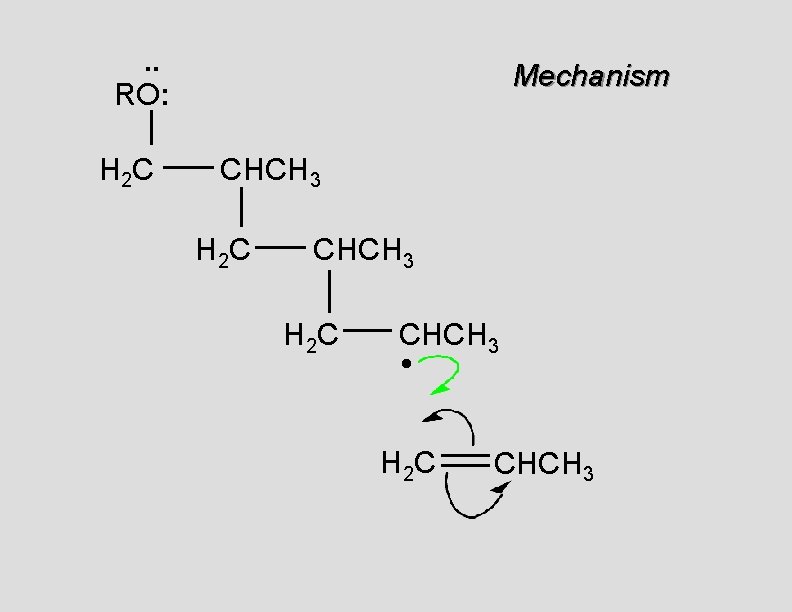
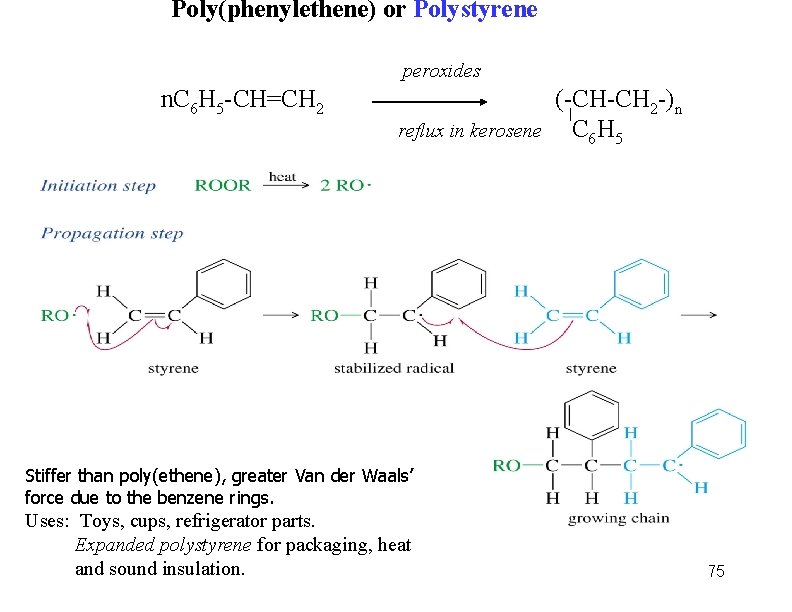
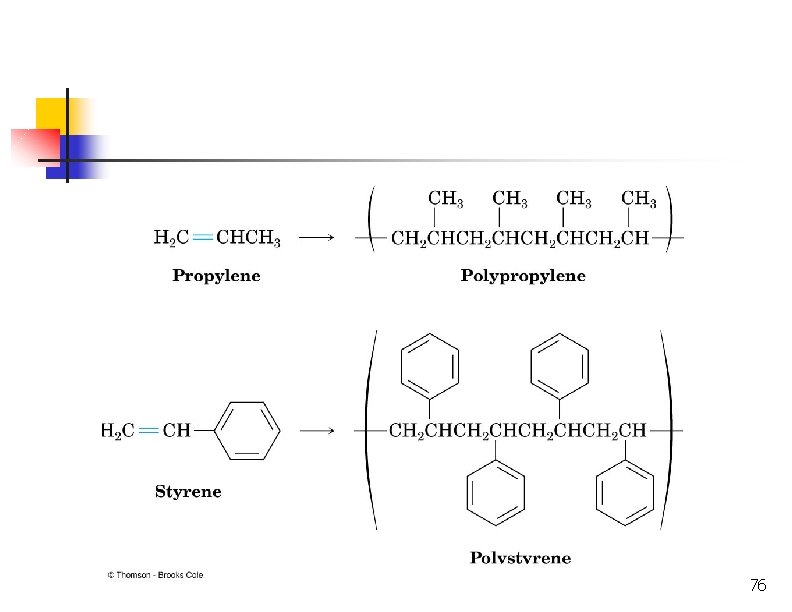
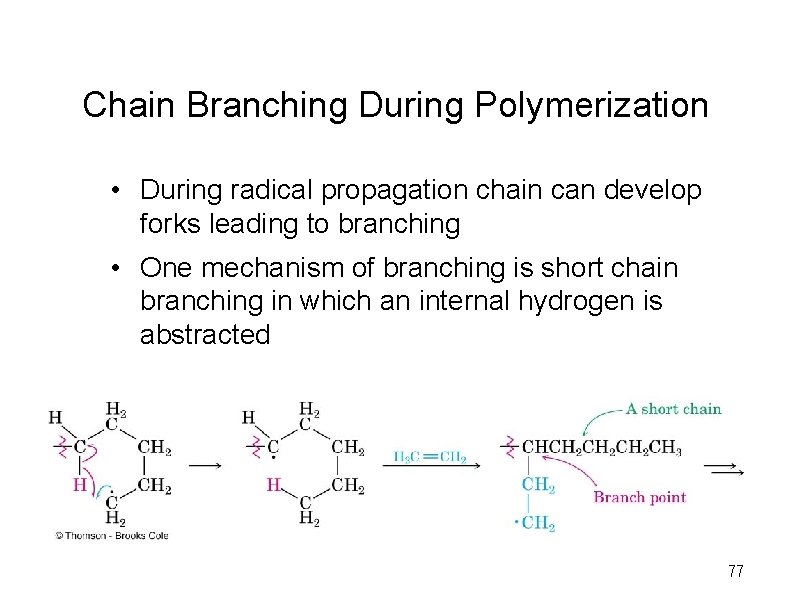
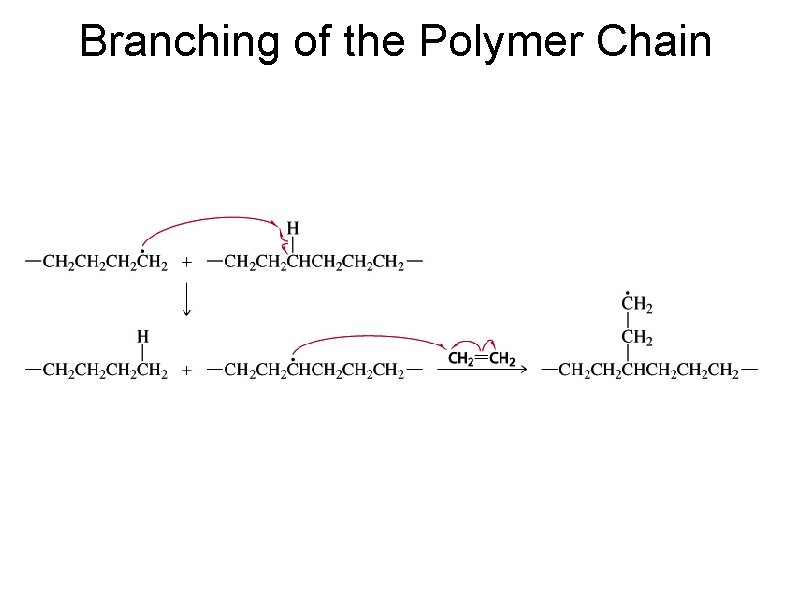
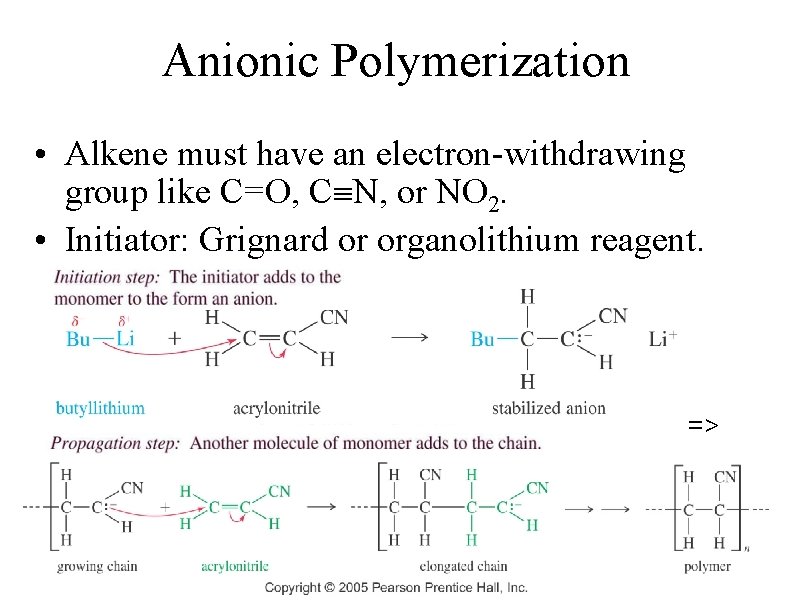
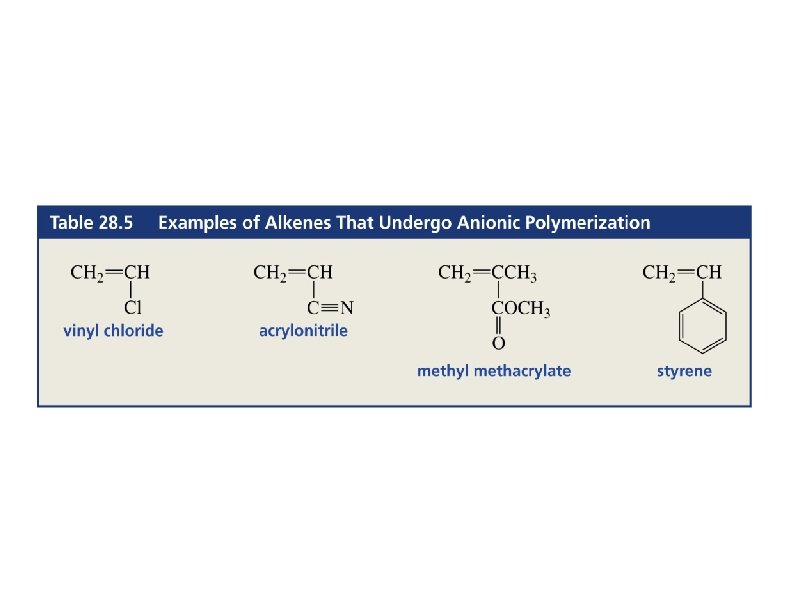
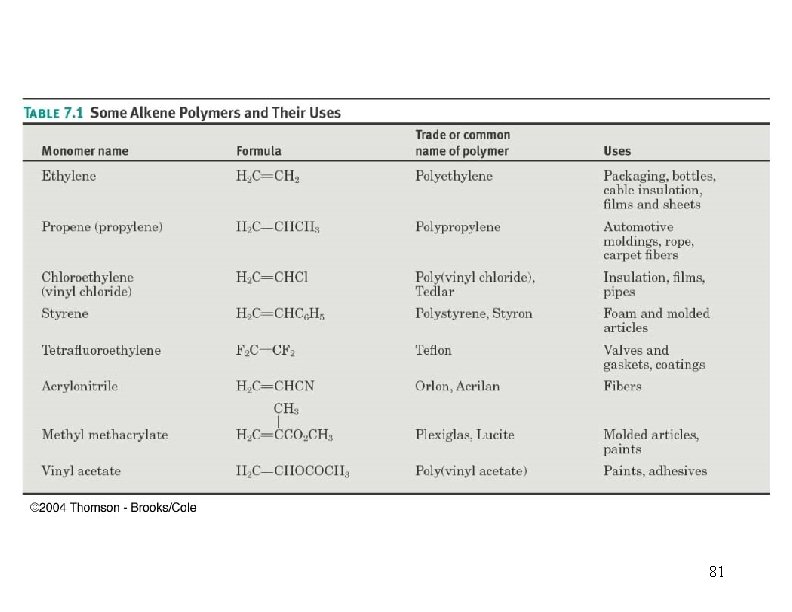
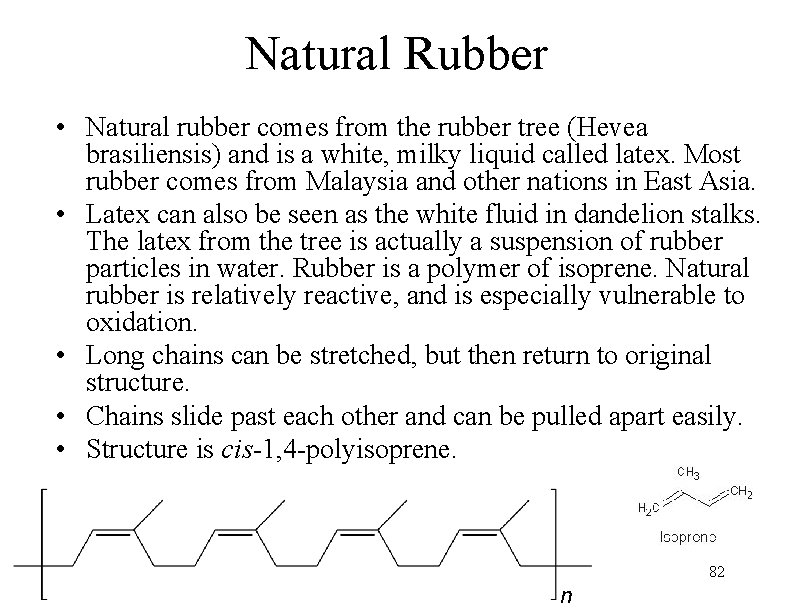
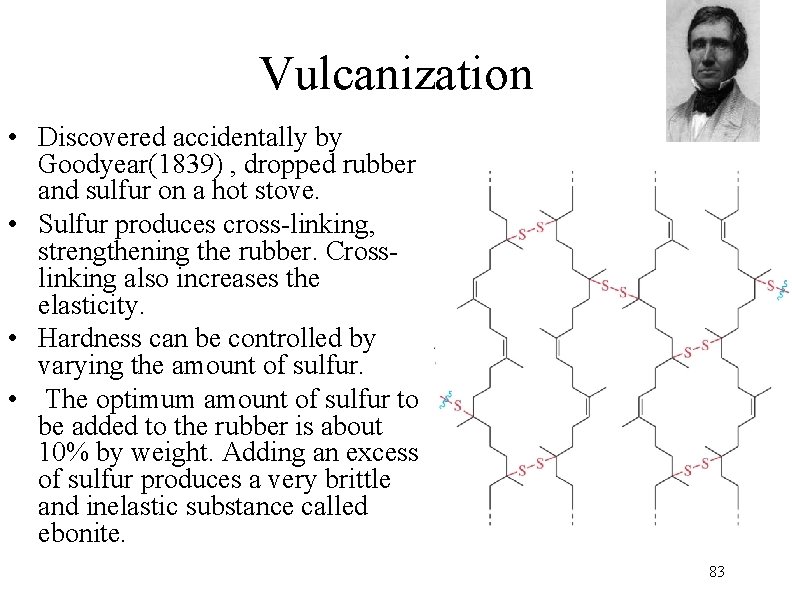
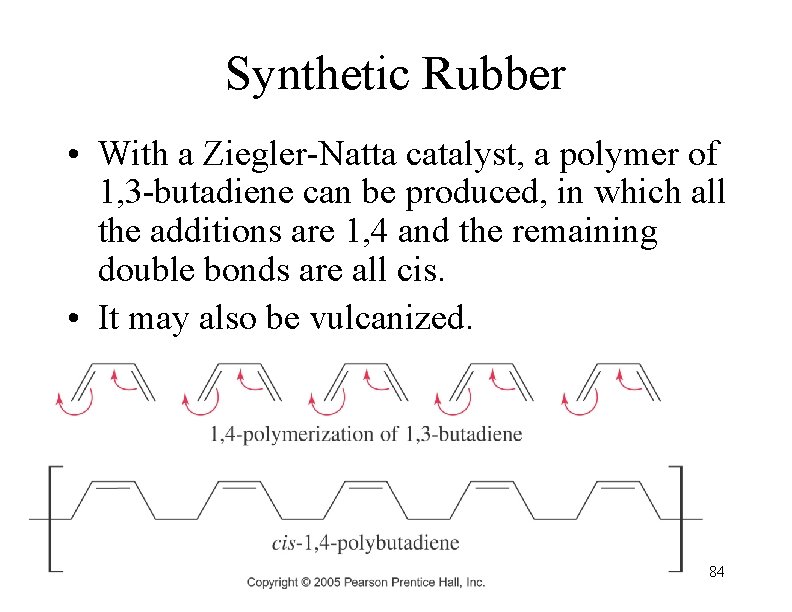
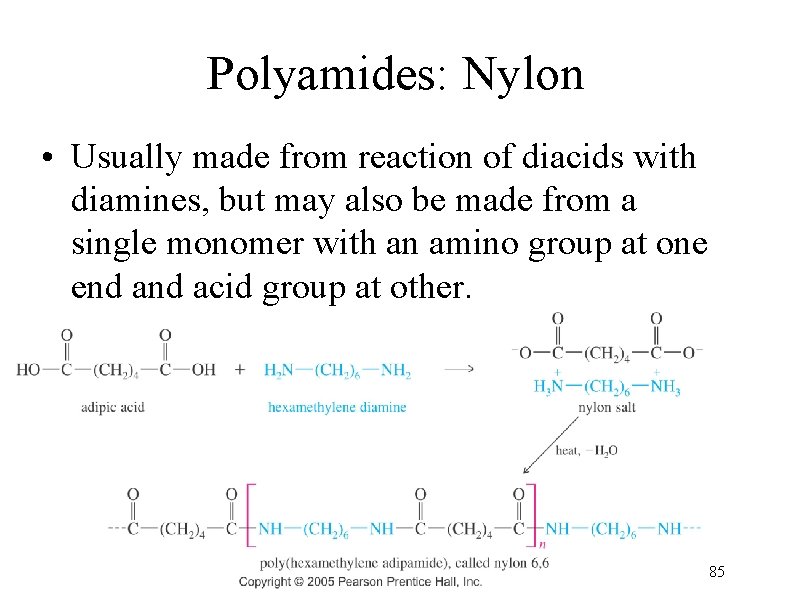
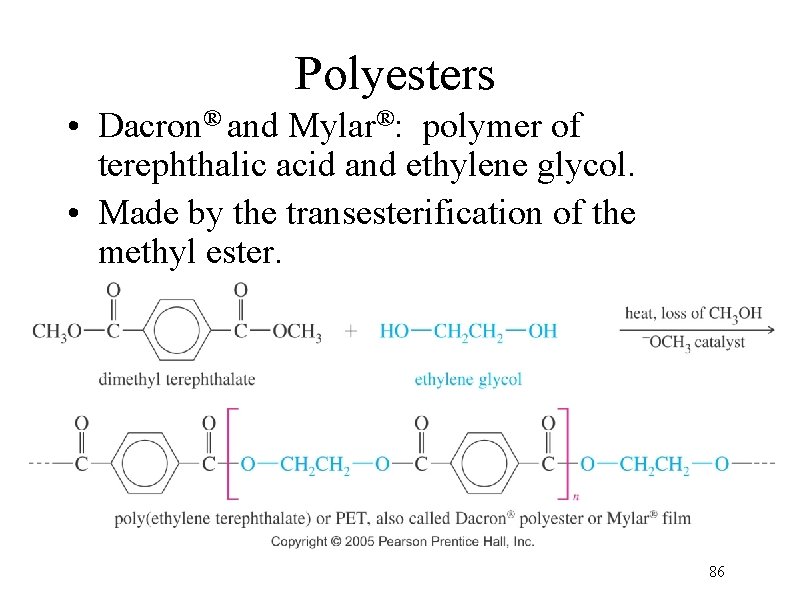
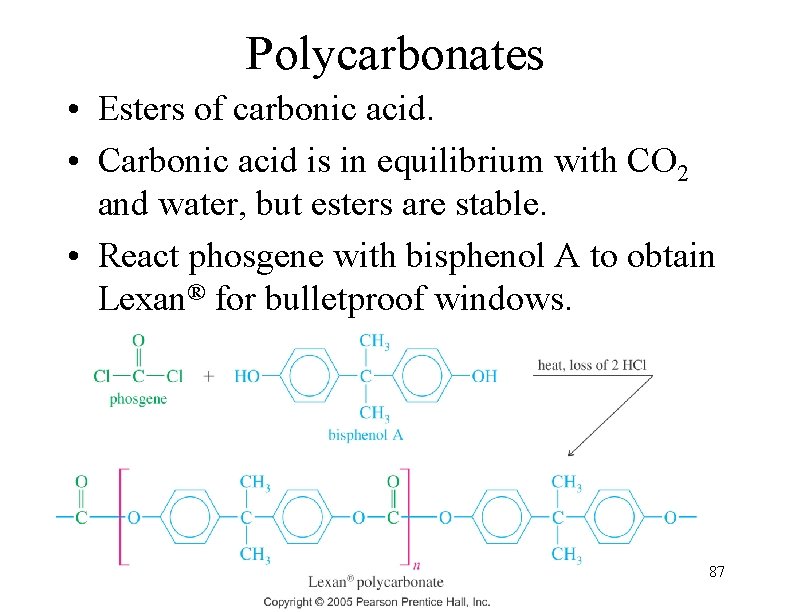
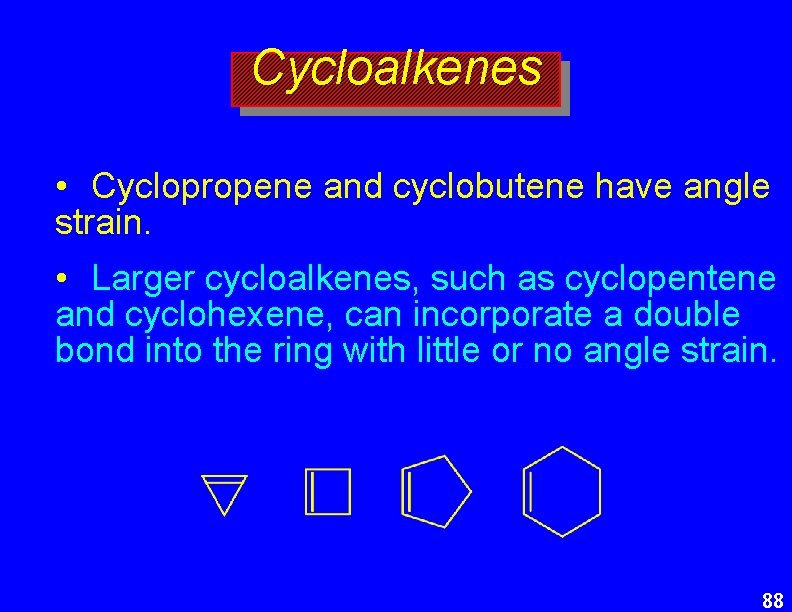
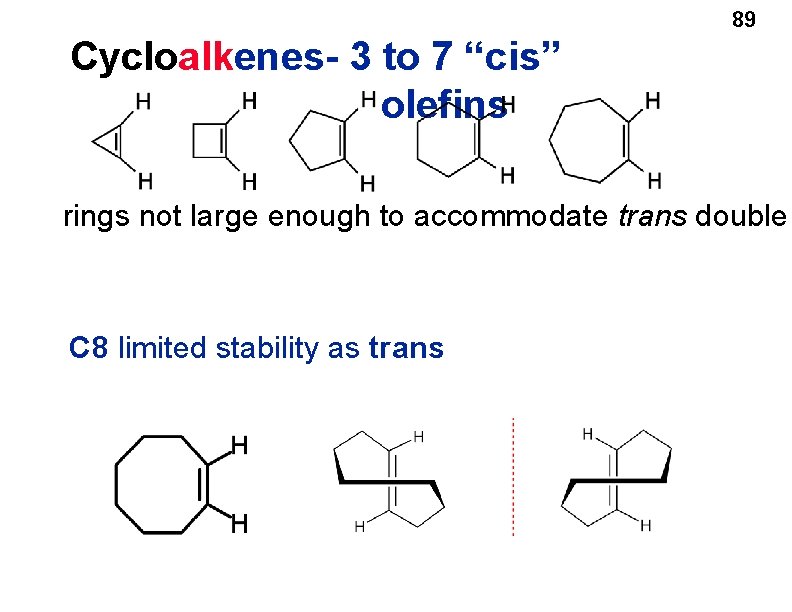
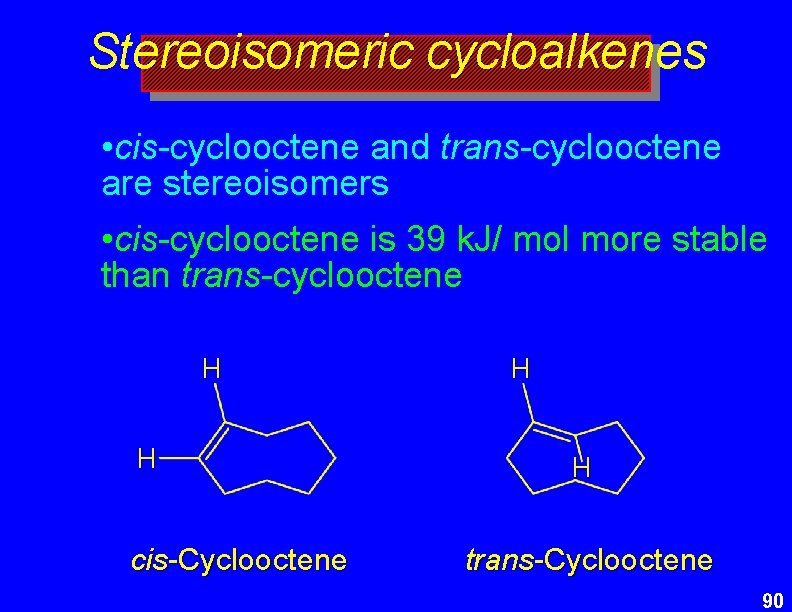
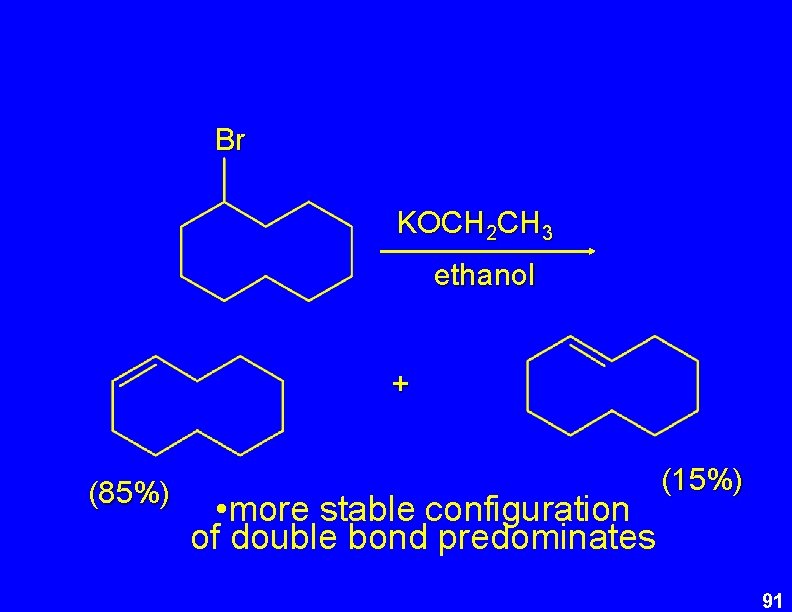
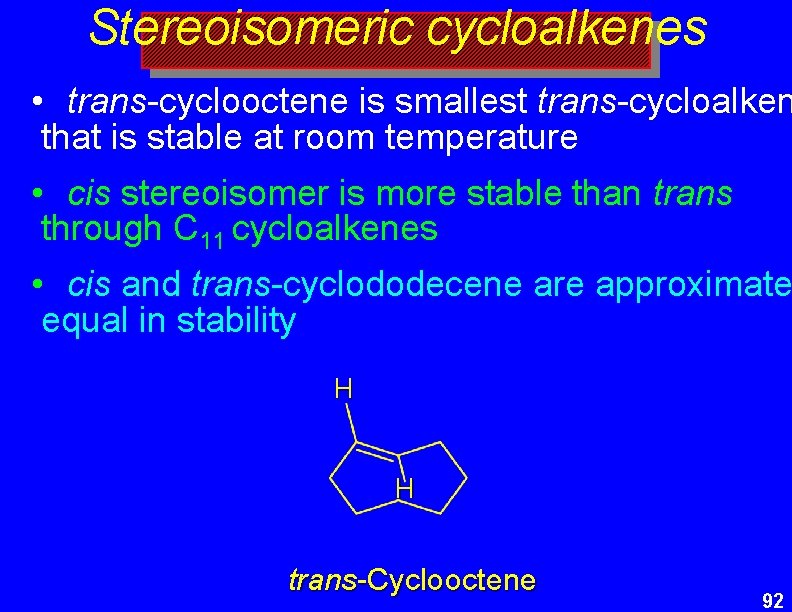
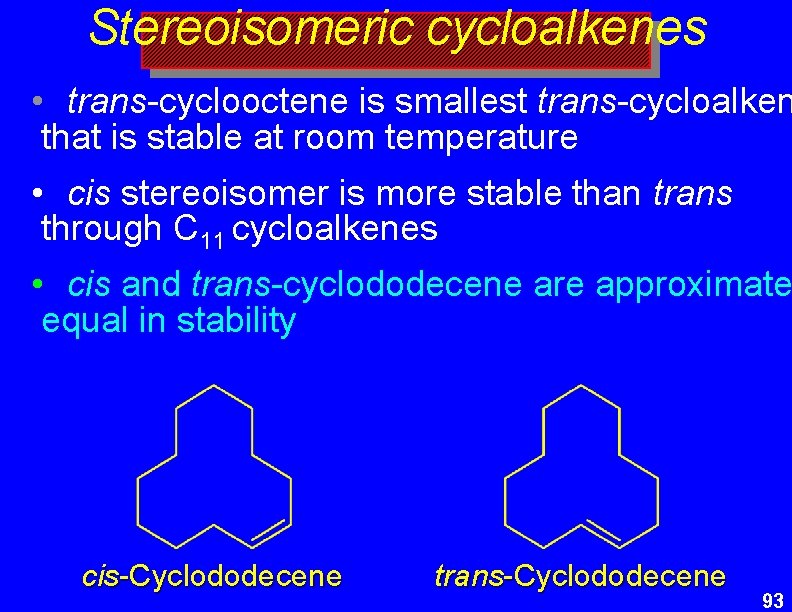
- Slides: 93
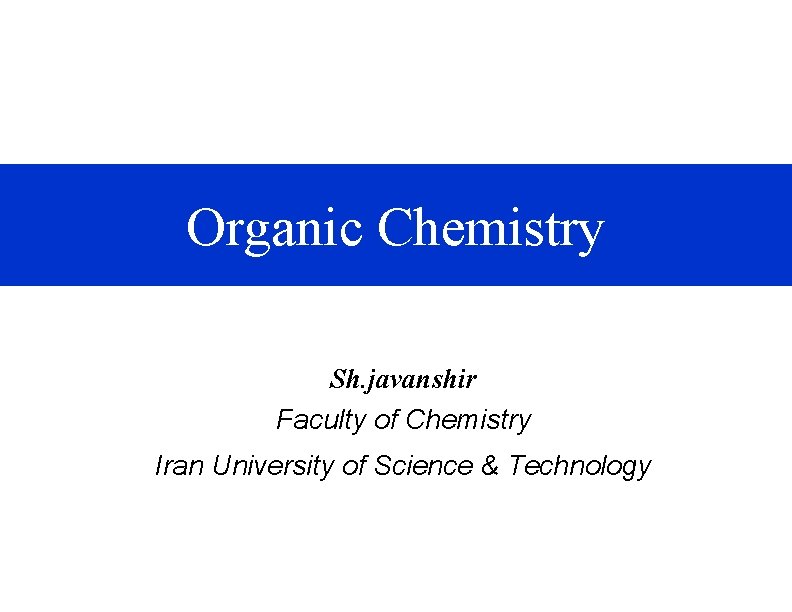
Organic Chemistry Sh. javanshir Faculty of Chemistry Iran University of Science & Technology
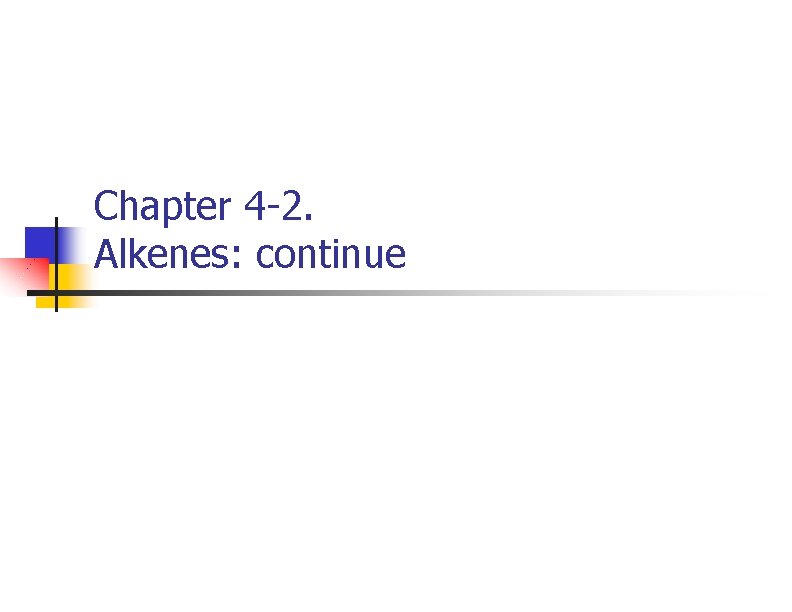
Chapter 4 -2. Alkenes: continue
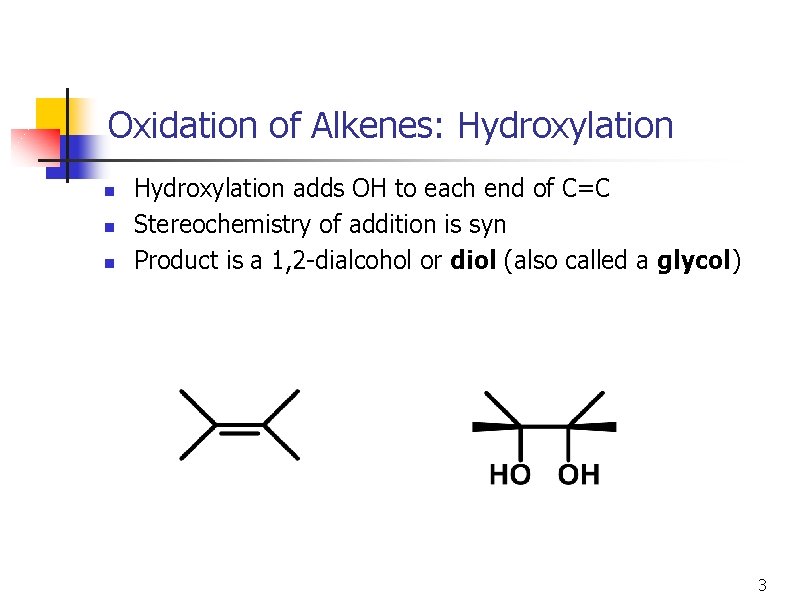
Oxidation of Alkenes: Hydroxylation n Hydroxylation adds OH to each end of C=C Stereochemistry of addition is syn Product is a 1, 2 -dialcohol or diol (also called a glycol) 3
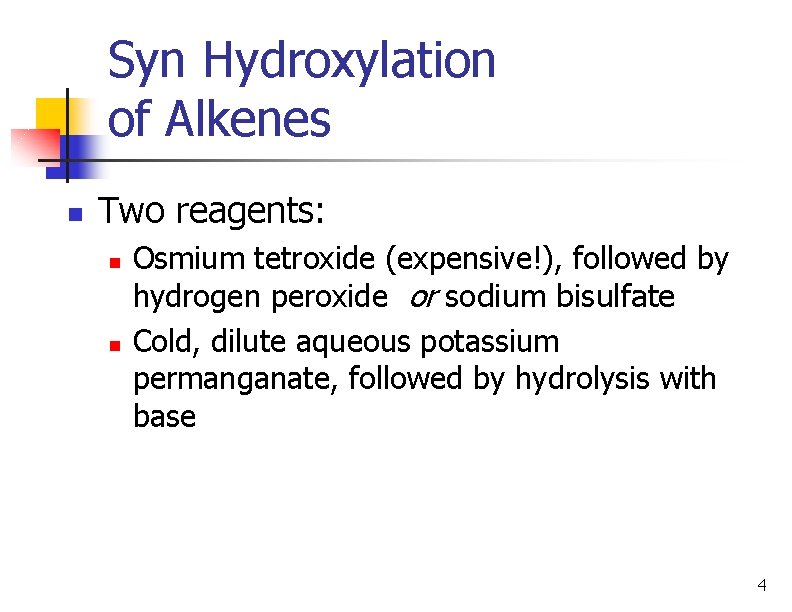
Syn Hydroxylation of Alkenes n Two reagents: n n Osmium tetroxide (expensive!), followed by hydrogen peroxide or sodium bisulfate Cold, dilute aqueous potassium permanganate, followed by hydrolysis with base 4
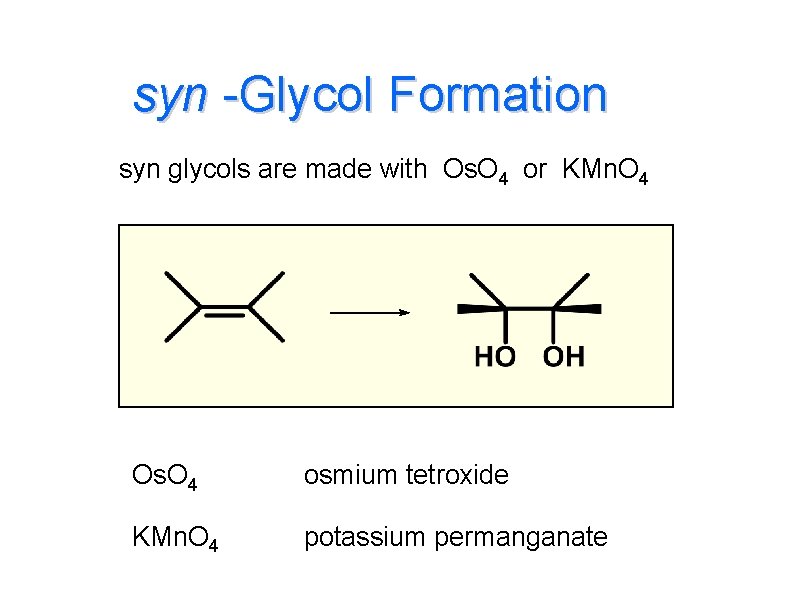
syn -Glycol Formation syn glycols are made with Os. O 4 or KMn. O 4 Os. O 4 osmium tetroxide KMn. O 4 potassium permanganate
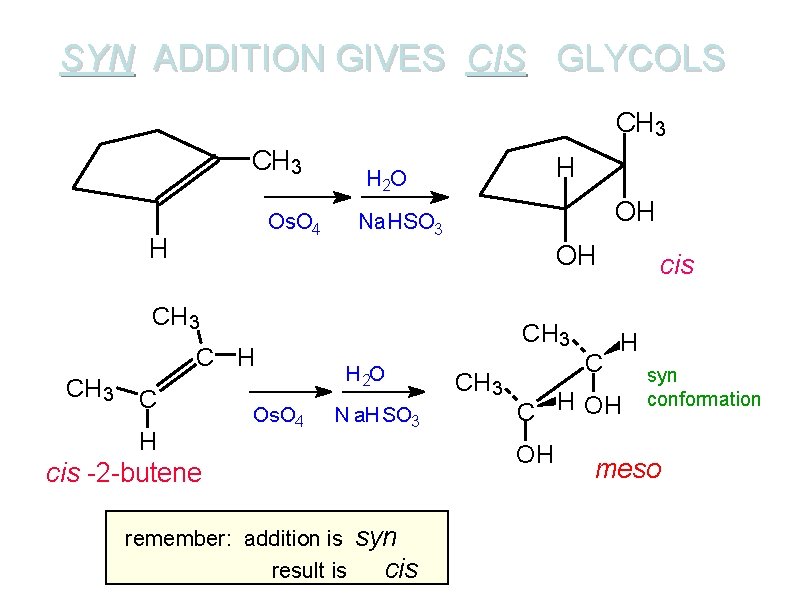
SYN ADDITION GIVES CIS GLYCOLS CH 3 Os. O 4 H H H 2 O OH Na. HSO 3 OH CH 3 C H Os. O 4 CH 3 H 2 O N a. HSO 3 syn cis H C H OH OH cis -2 -butene remember: addition is result is CH 3 C cis syn conformation meso
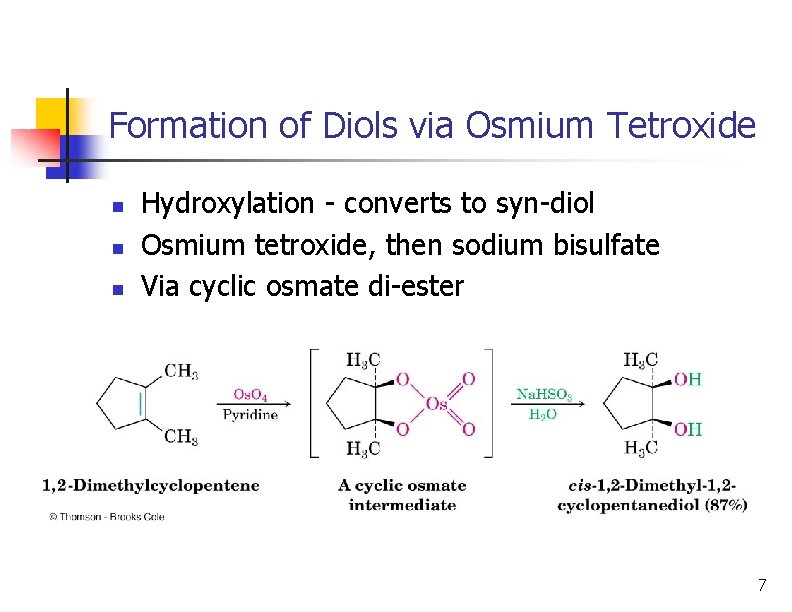
Formation of Diols via Osmium Tetroxide n n n Hydroxylation - converts to syn-diol Osmium tetroxide, then sodium bisulfate Via cyclic osmate di-ester 7
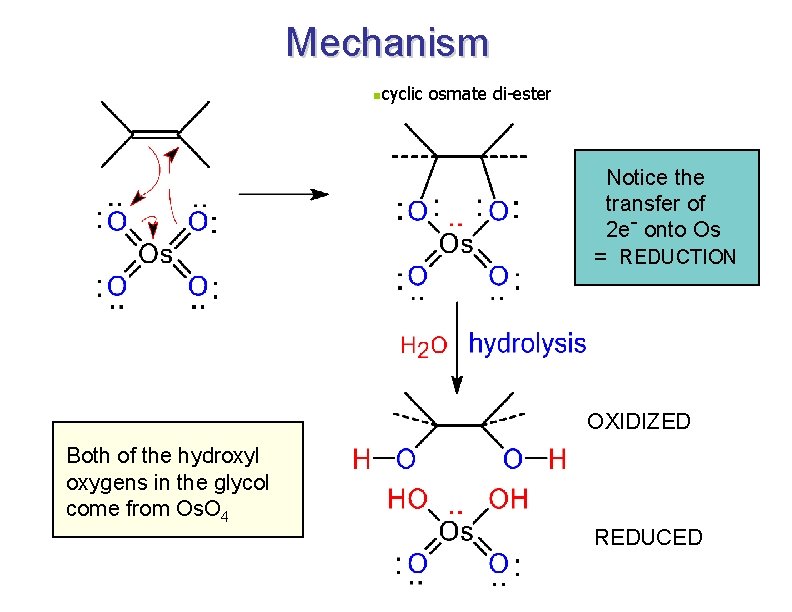
Mechanism ncyclic osmate di-ester Notice the transfer of 2 e- onto Os = REDUCTION OXIDIZED Both of the hydroxyl oxygens in the glycol come from Os. O 4 REDUCED
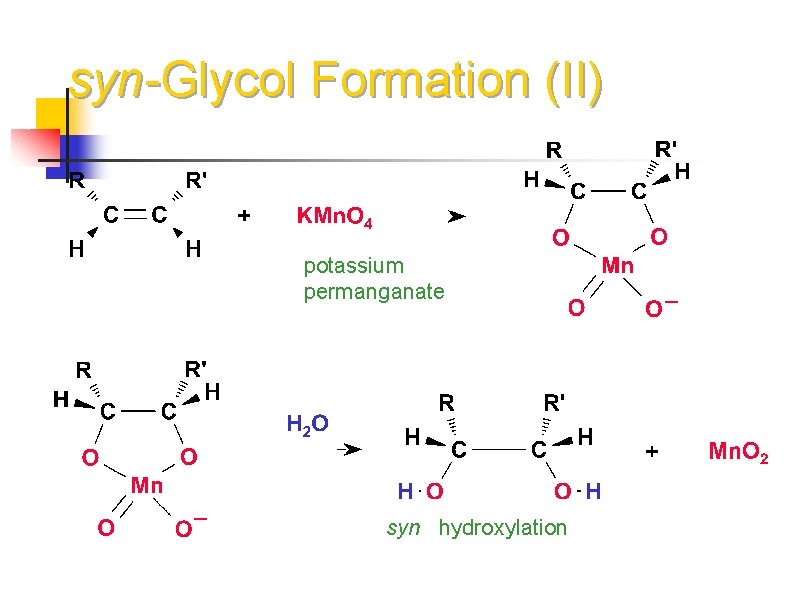
syn-Glycol Formation (II) potassium permanganate syn hydroxylation
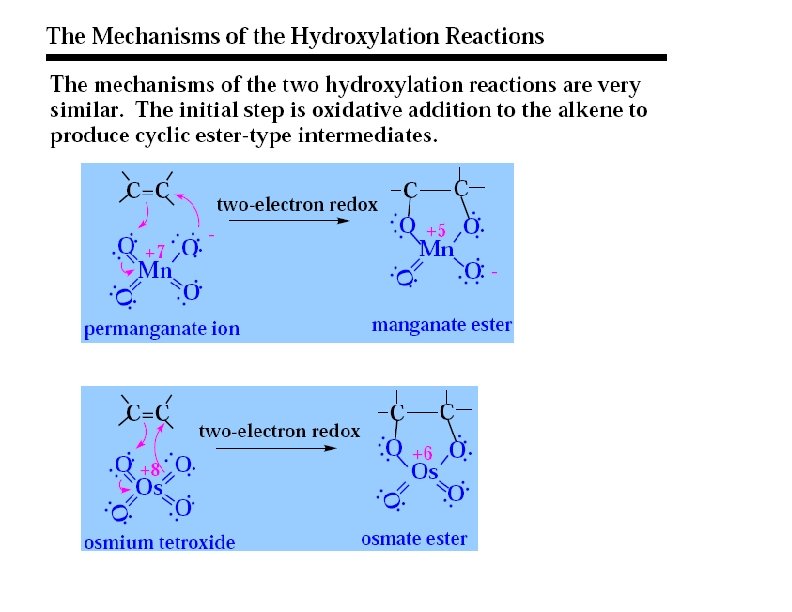
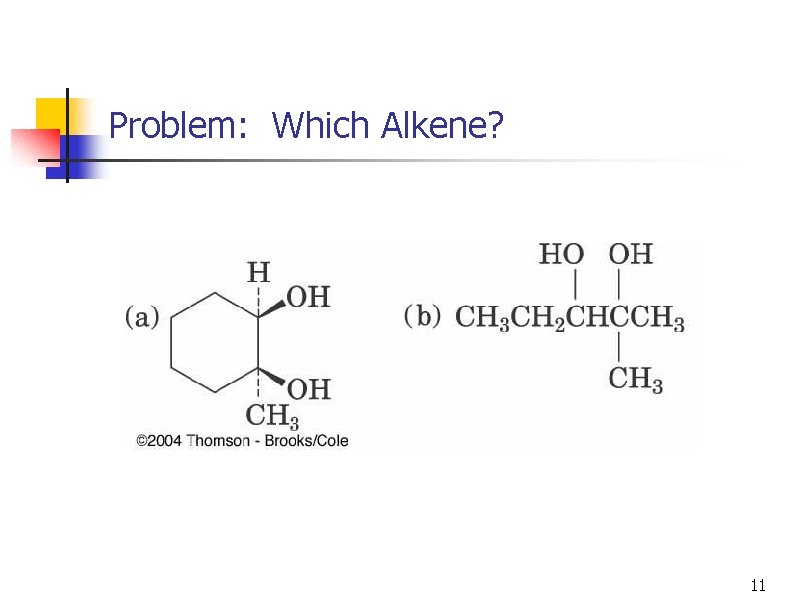
Problem: Which Alkene? 11
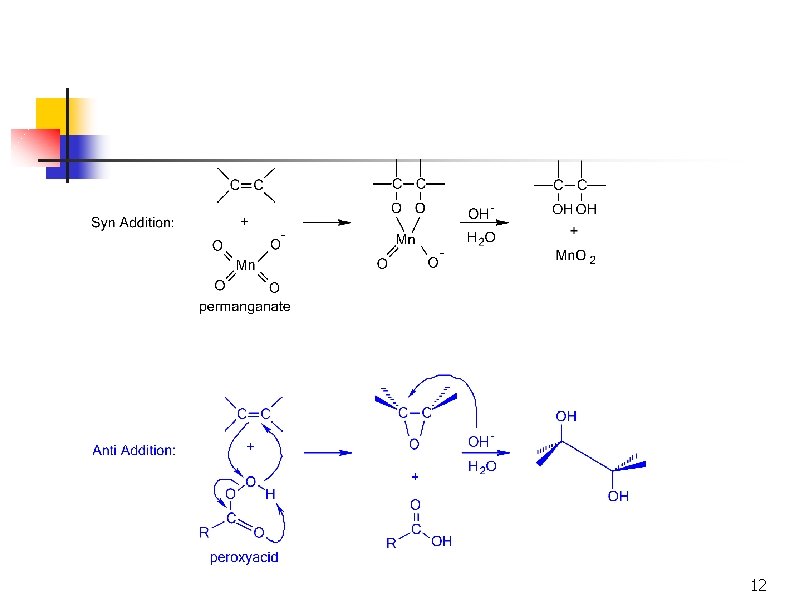
12
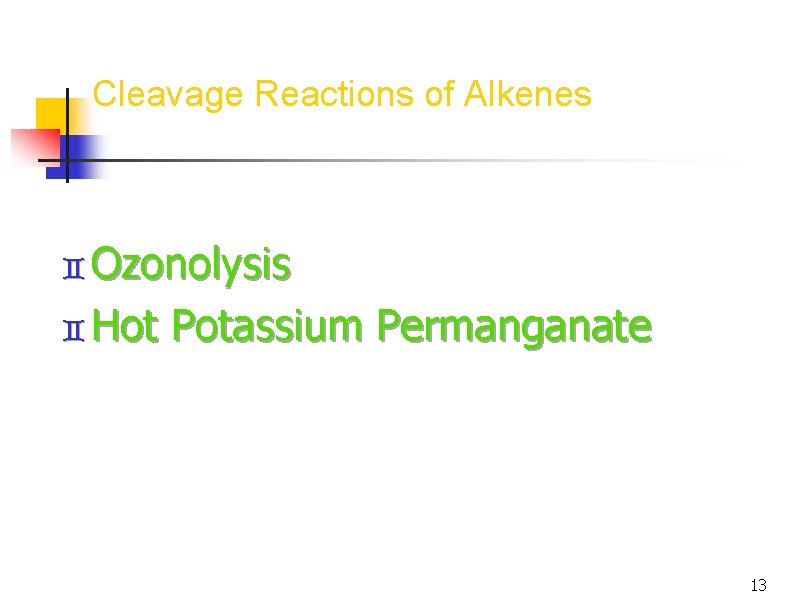
Cleavage Reactions of Alkenes Ozonolysis Hot Potassium Permanganate 13
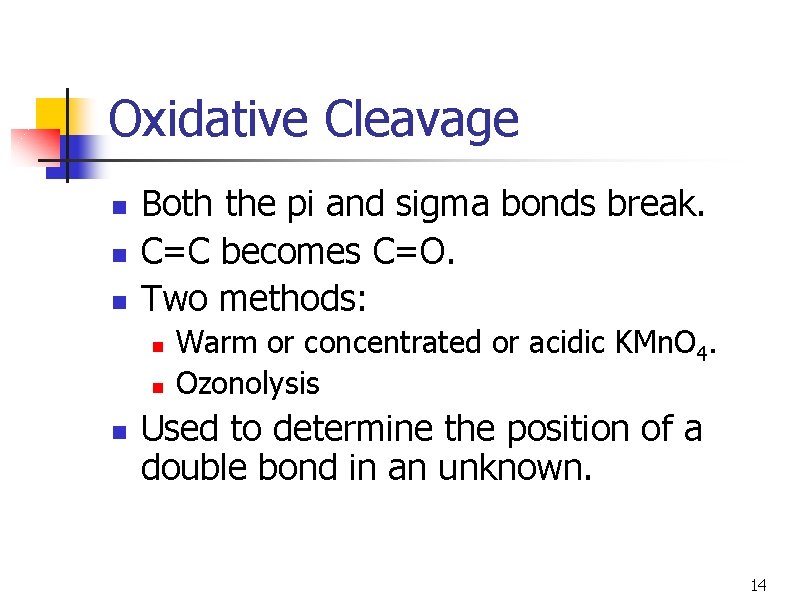
Oxidative Cleavage n n n Both the pi and sigma bonds break. C=C becomes C=O. Two methods: n n n Warm or concentrated or acidic KMn. O 4. Ozonolysis Used to determine the position of a double bond in an unknown. 14
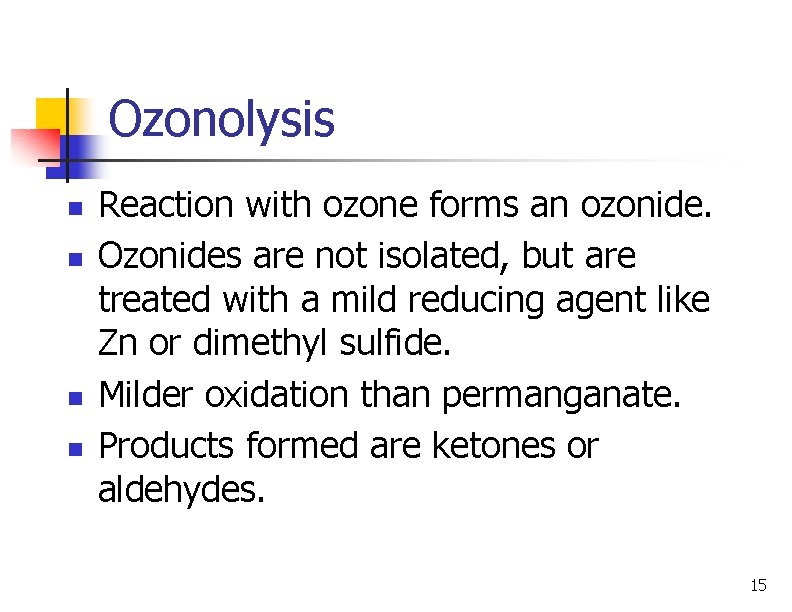
Ozonolysis n n Reaction with ozone forms an ozonide. Ozonides are not isolated, but are treated with a mild reducing agent like Zn or dimethyl sulfide. Milder oxidation than permanganate. Products formed are ketones or aldehydes. 15
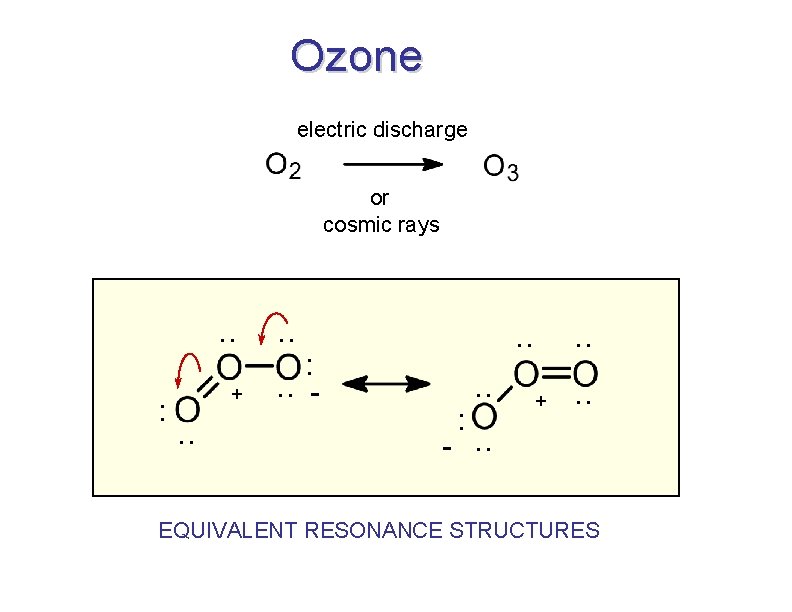
Ozone electric discharge or cosmic rays . . : + . . : . . - . . : . . + . . -. . EQUIVALENT RESONANCE STRUCTURES
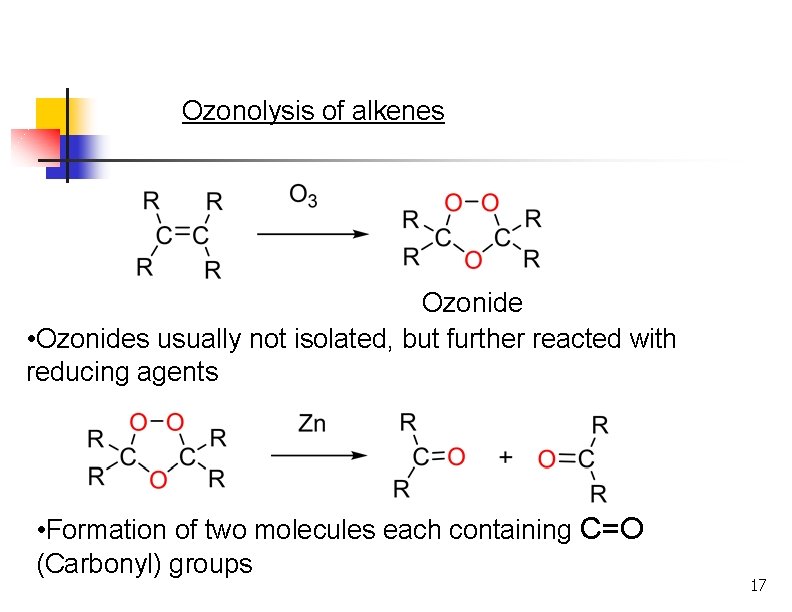
Ozonolysis of alkenes Ozonide • Ozonides usually not isolated, but further reacted with reducing agents • Formation of two molecules each containing C=O (Carbonyl) groups 17
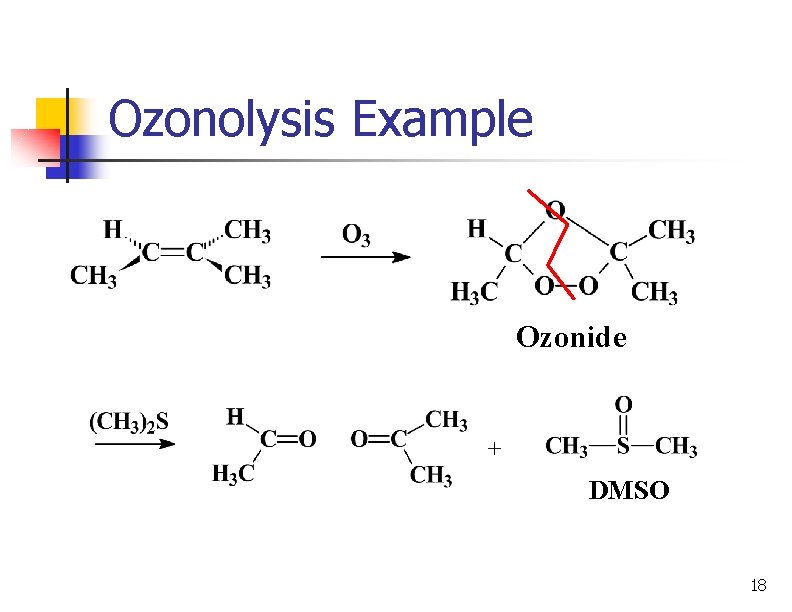
Ozonolysis Example Ozonide DMSO 18
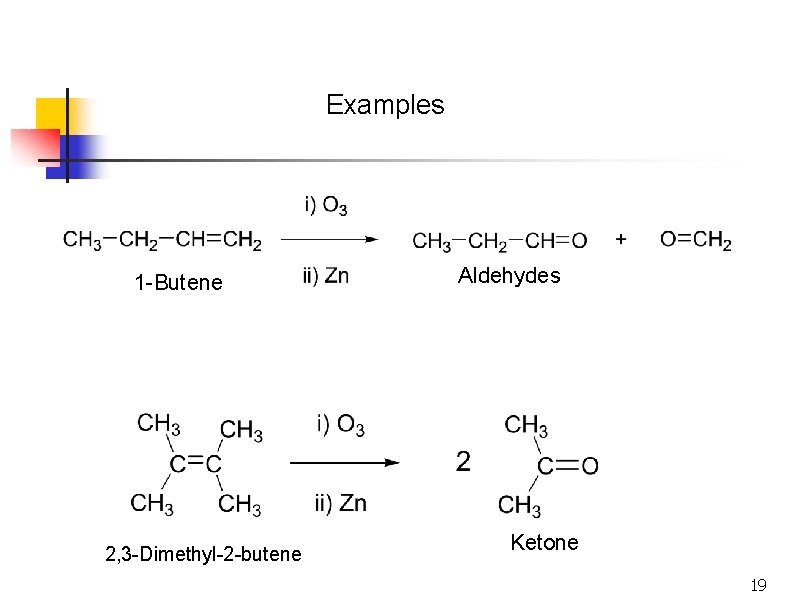
Examples 1 -Butene 2, 3 -Dimethyl-2 -butene Aldehydes Ketone 19
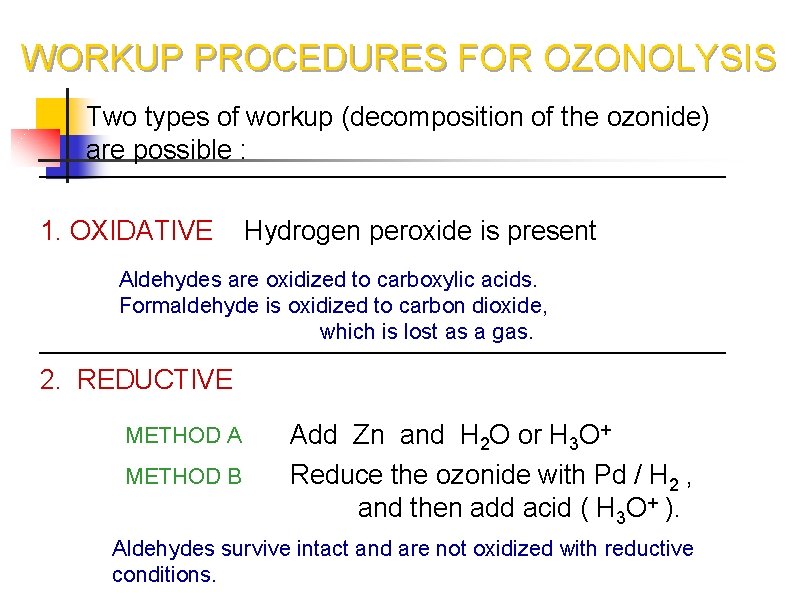
WORKUP PROCEDURES FOR OZONOLYSIS Two types of workup (decomposition of the ozonide) are possible : 1. OXIDATIVE Hydrogen peroxide is present Aldehydes are oxidized to carboxylic acids. Formaldehyde is oxidized to carbon dioxide, which is lost as a gas. 2. REDUCTIVE METHOD A Add Zn and H 2 O or H 3 O+ METHOD B Reduce the ozonide with Pd / H 2 , and then add acid ( H 3 O+ ). Aldehydes survive intact and are not oxidized with reductive conditions.
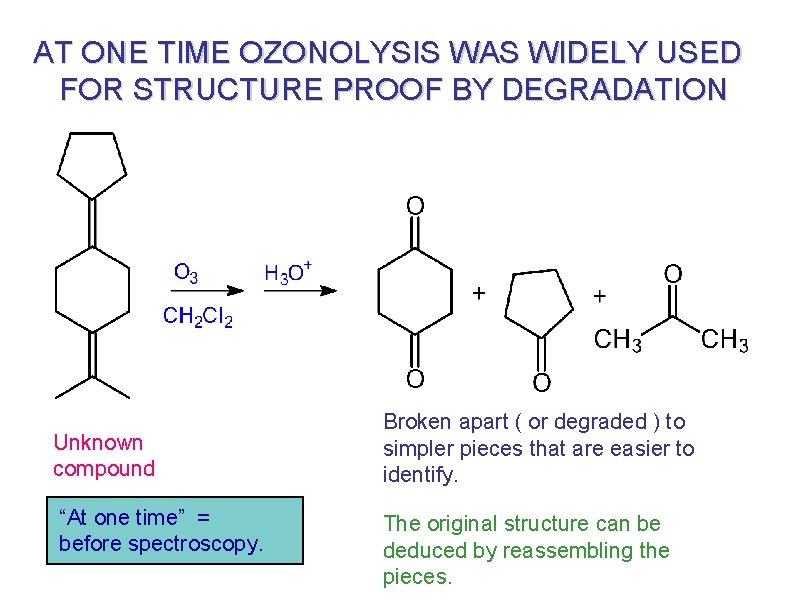
AT ONE TIME OZONOLYSIS WAS WIDELY USED FOR STRUCTURE PROOF BY DEGRADATION Unknown compound “At one time” = before spectroscopy. Broken apart ( or degraded ) to simpler pieces that are easier to identify. The original structure can be deduced by reassembling the pieces.
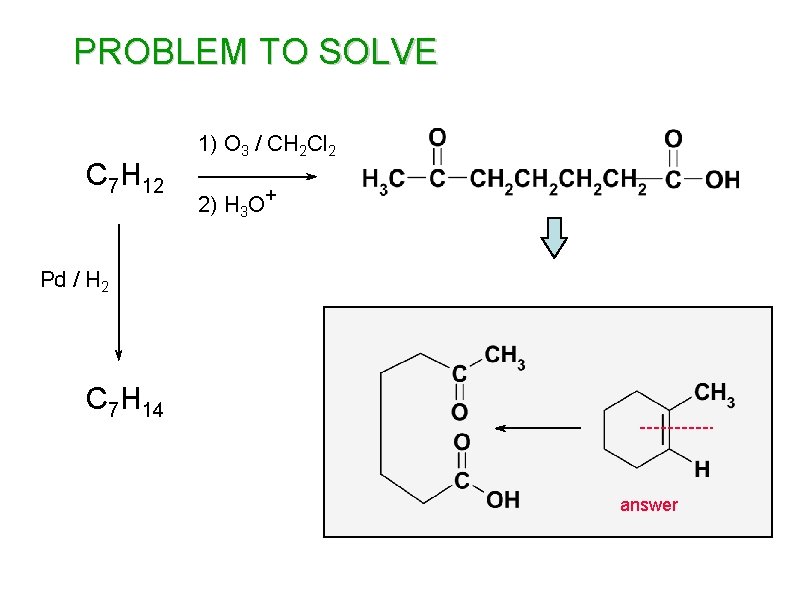
PROBLEM TO SOLVE C 7 H 12 1) O 3 / CH 2 Cl 2 2) H 3 O+ Pd / H 2 C 7 H 14 answer
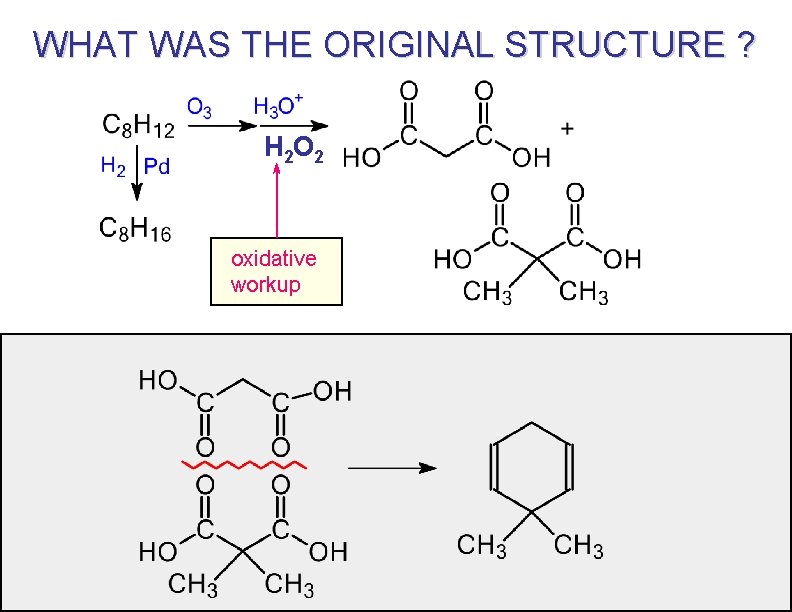
WHAT WAS THE ORIGINAL STRUCTURE ? H 2 O 2 oxidative workup
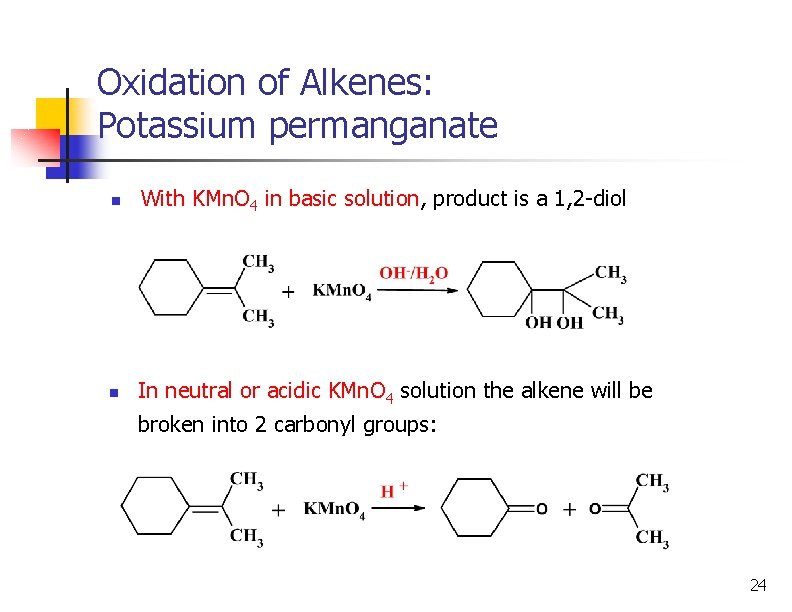
Oxidation of Alkenes: Potassium permanganate n With KMn. O 4 in basic solution, product is a 1, 2 -diol n In neutral or acidic KMn. O 4 solution the alkene will be broken into 2 carbonyl groups: 24
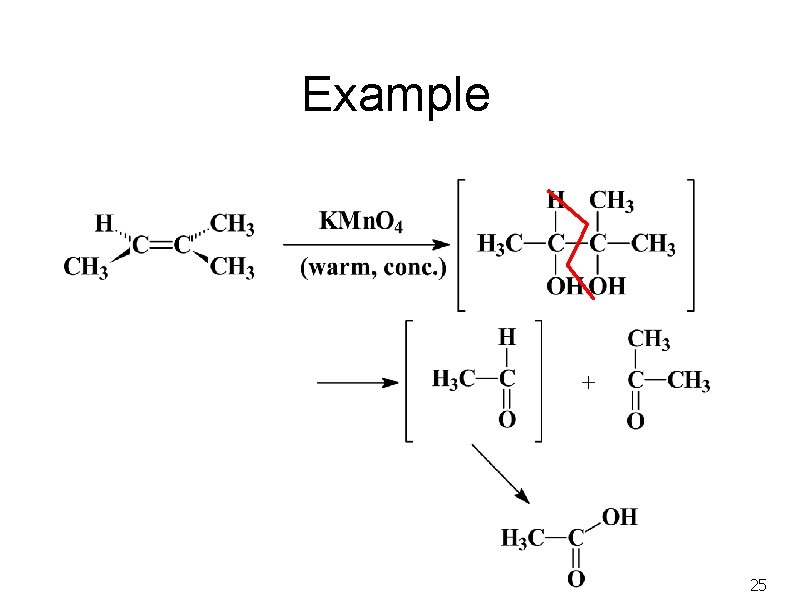
Example 25
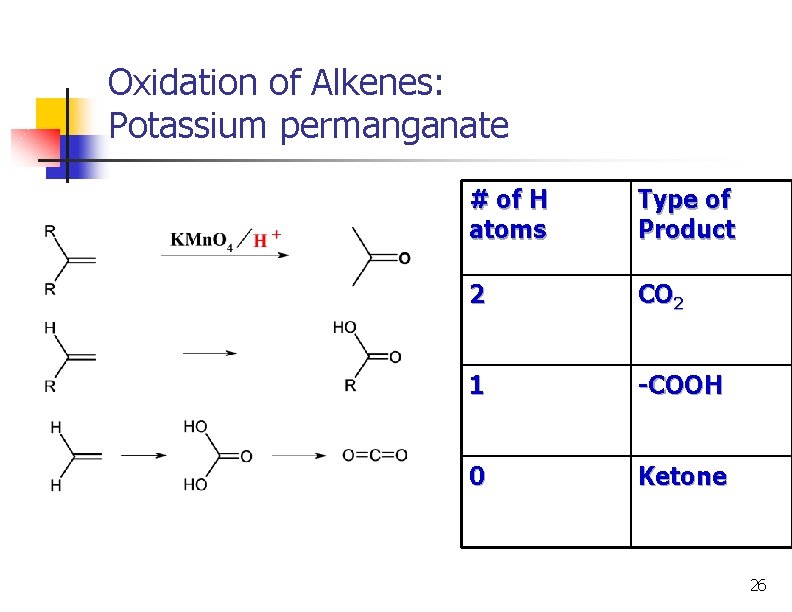
Oxidation of Alkenes: Potassium permanganate # of H atoms Type of Product 2 CO 2 1 -COOH 0 Ketone 26
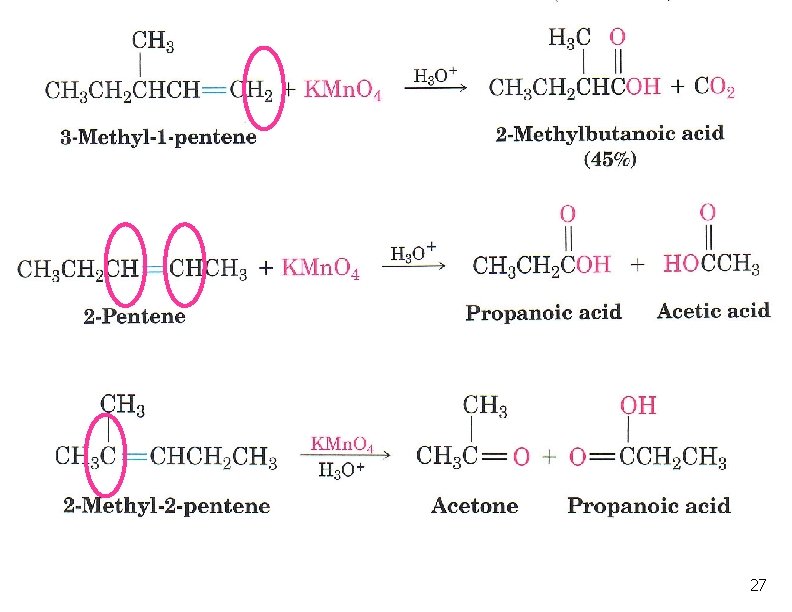
27
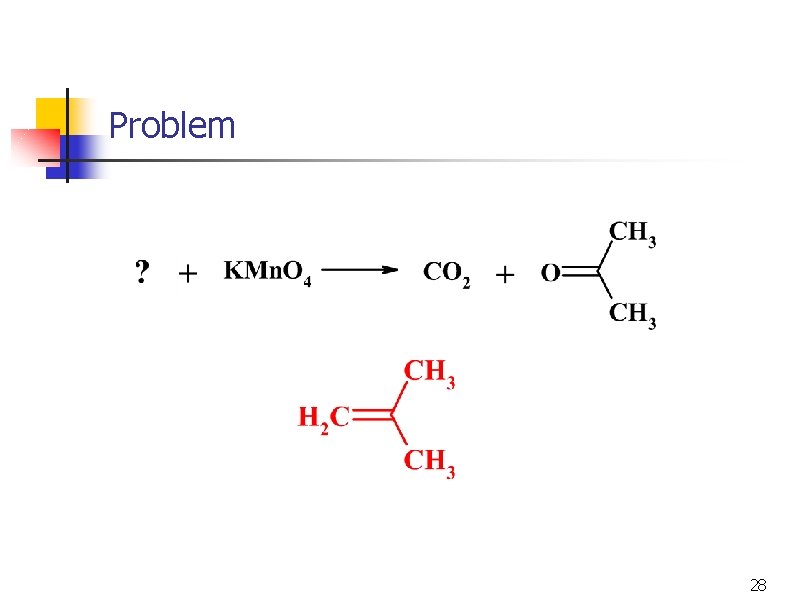
Problem 28
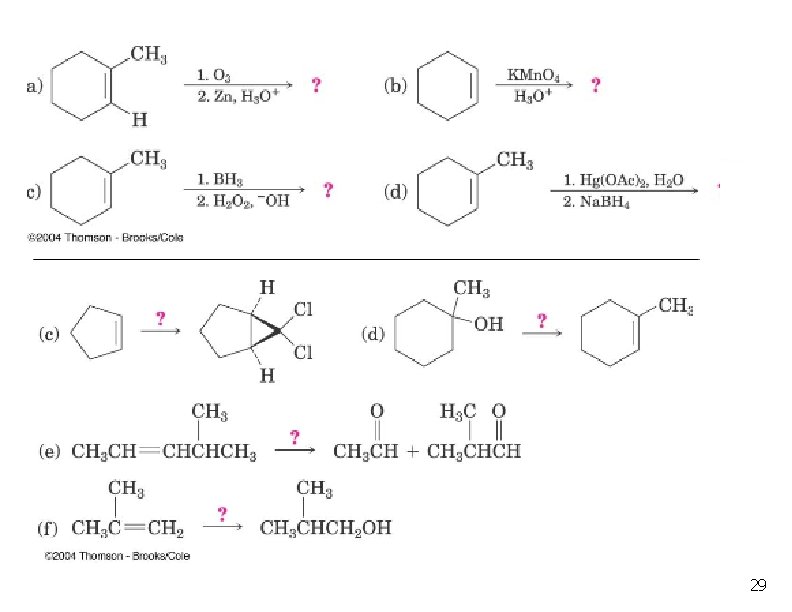
29
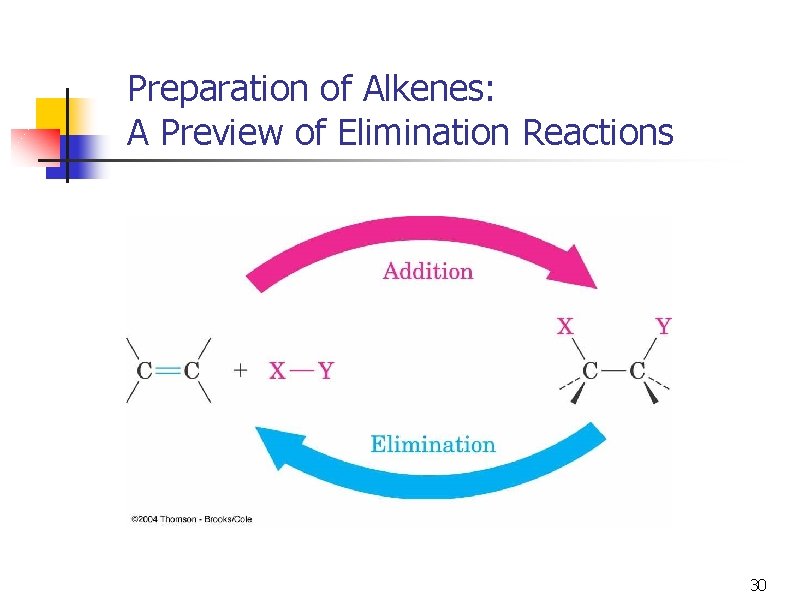
Preparation of Alkenes: A Preview of Elimination Reactions 30
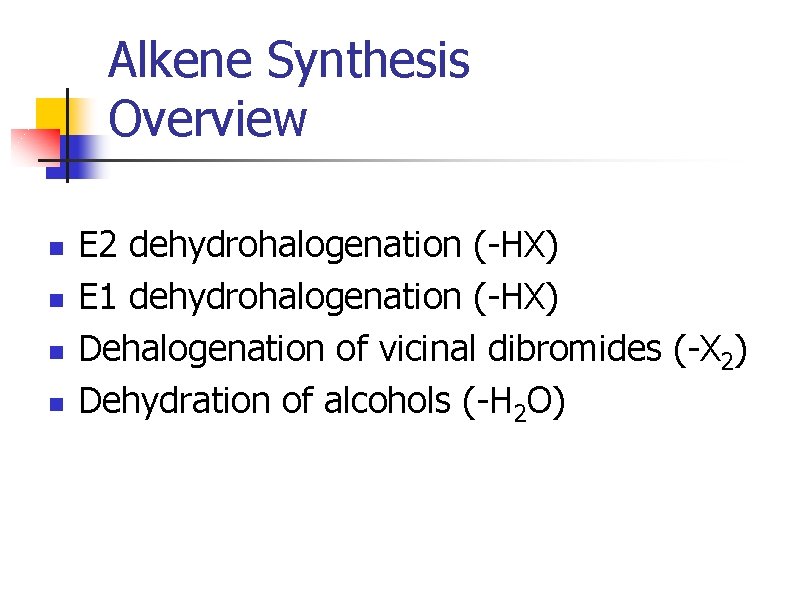
Alkene Synthesis Overview n n E 2 dehydrohalogenation (-HX) E 1 dehydrohalogenation (-HX) Dehalogenation of vicinal dibromides (-X 2) Dehydration of alcohols (-H 2 O)
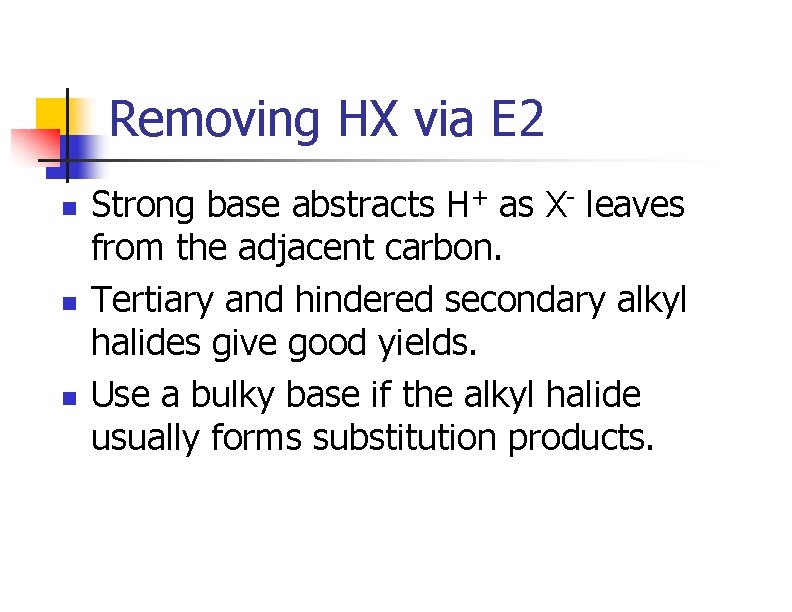
Removing HX via E 2 n n n Strong base abstracts H+ as X- leaves from the adjacent carbon. Tertiary and hindered secondary alkyl halides give good yields. Use a bulky base if the alkyl halide usually forms substitution products.
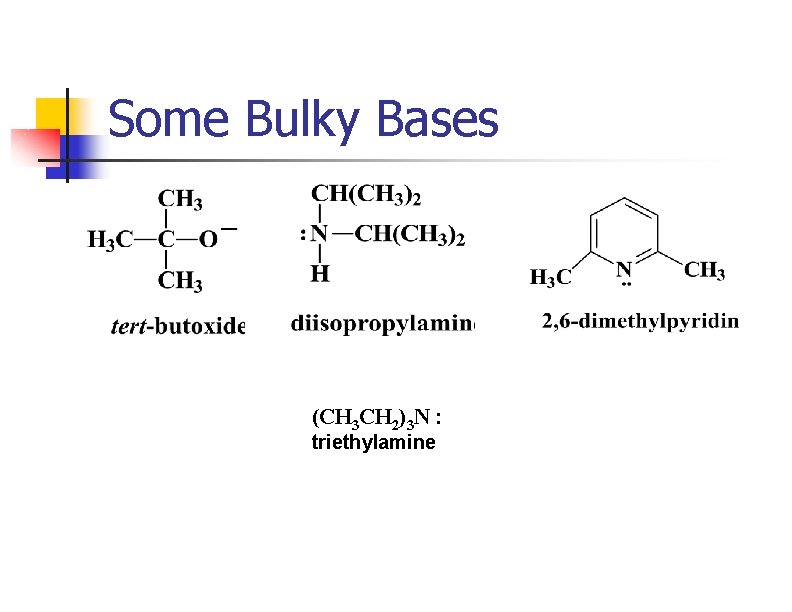
Some Bulky Bases (CH 3 CH 2)3 N : triethylamine
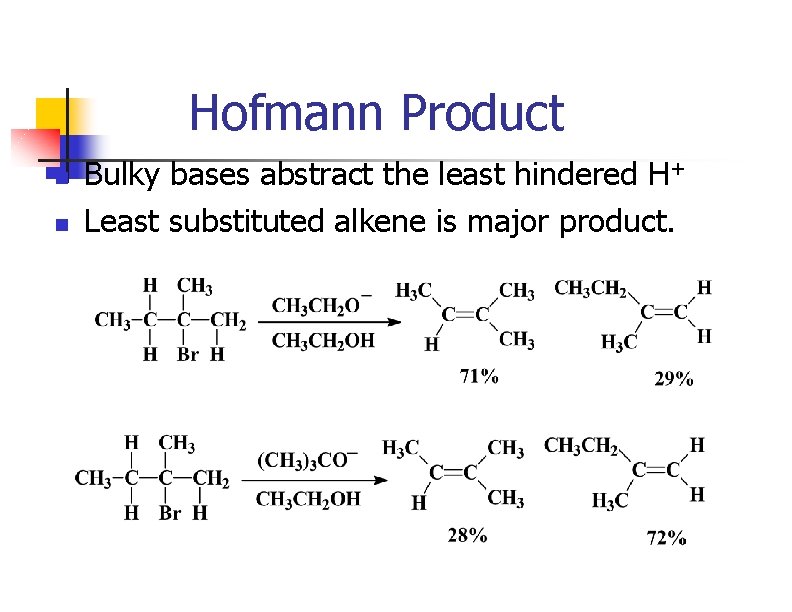
Hofmann Product n n Bulky bases abstract the least hindered H+ Least substituted alkene is major product.
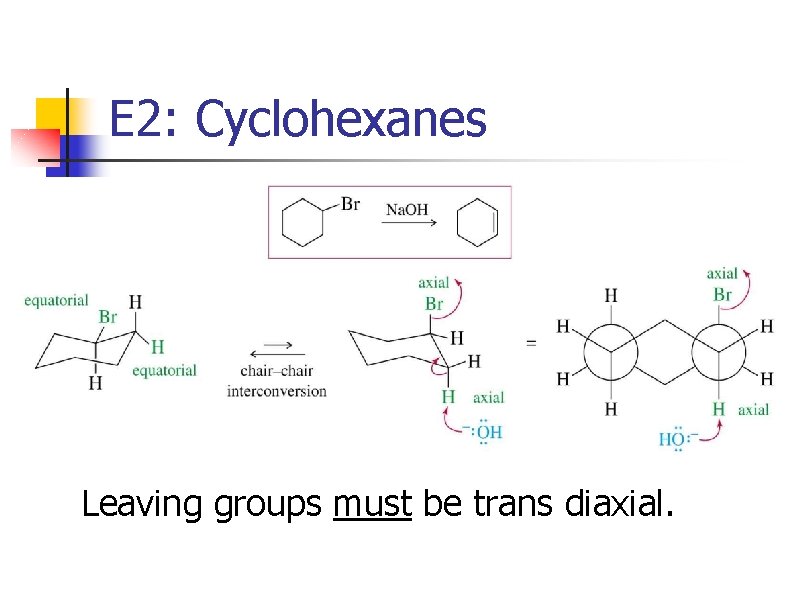
E 2: Cyclohexanes Leaving groups must be trans diaxial.
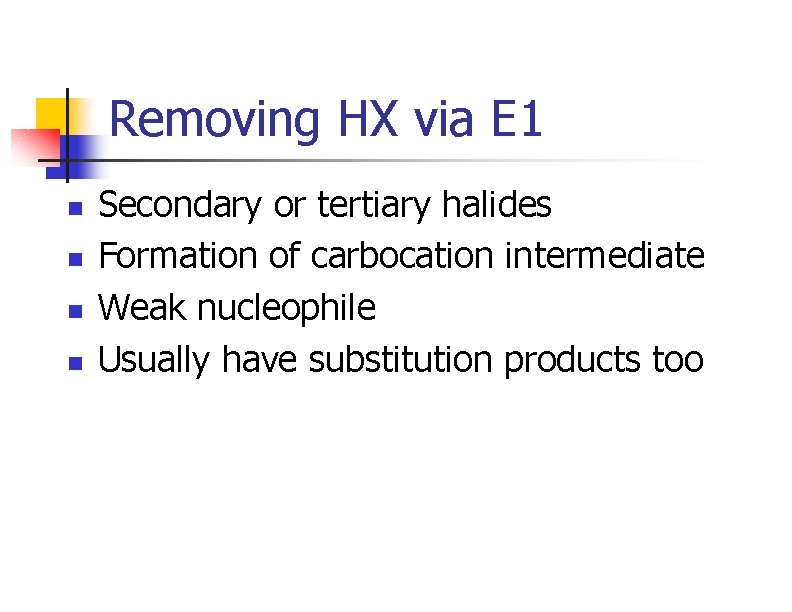
Removing HX via E 1 n n Secondary or tertiary halides Formation of carbocation intermediate Weak nucleophile Usually have substitution products too
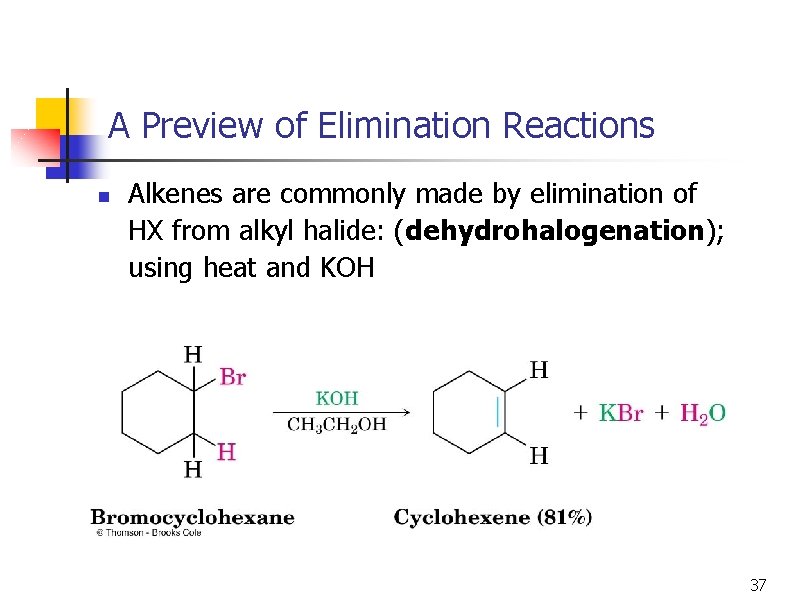
A Preview of Elimination Reactions n Alkenes are commonly made by elimination of HX from alkyl halide: (dehydrohalogenation); using heat and KOH 37
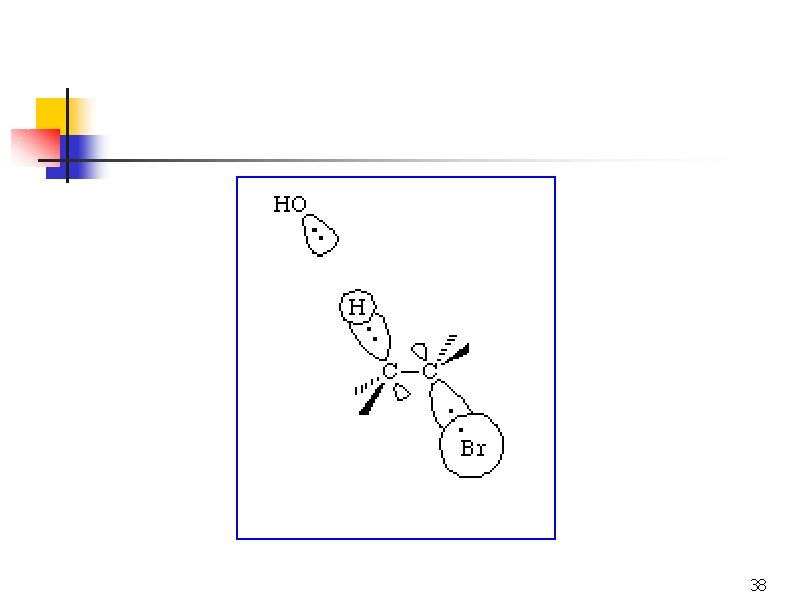
38
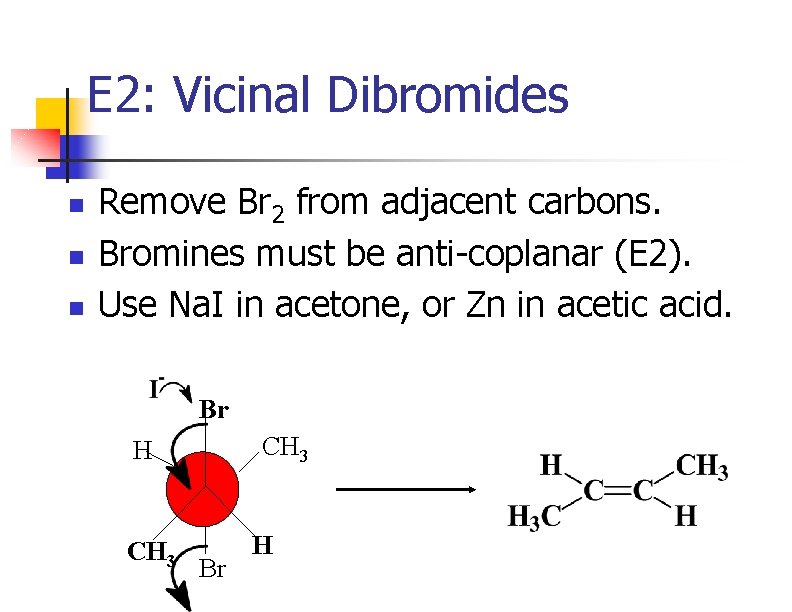
E 2: Vicinal Dibromides n n n Remove Br 2 from adjacent carbons. Bromines must be anti-coplanar (E 2). Use Na. I in acetone, or Zn in acetic acid. Br CH 3 H CH 3 Br H
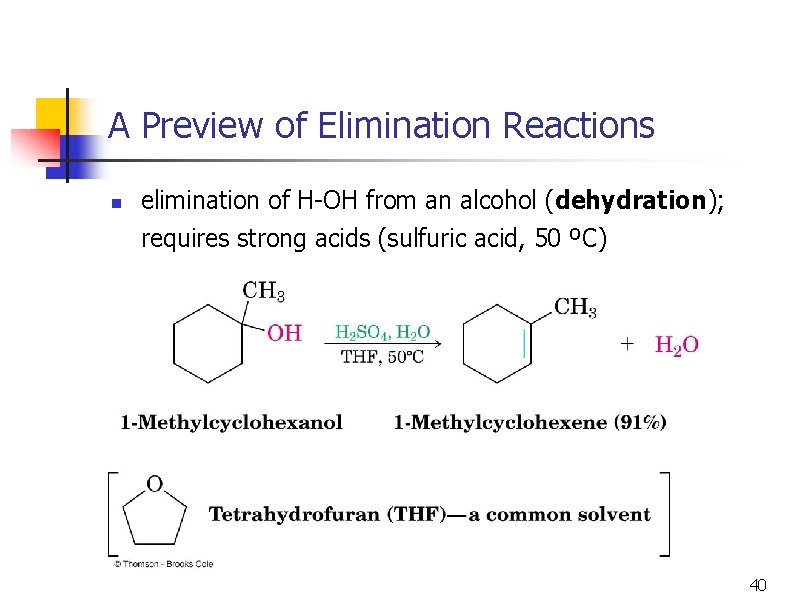
A Preview of Elimination Reactions n elimination of H-OH from an alcohol (dehydration); requires strong acids (sulfuric acid, 50 ºC) 40
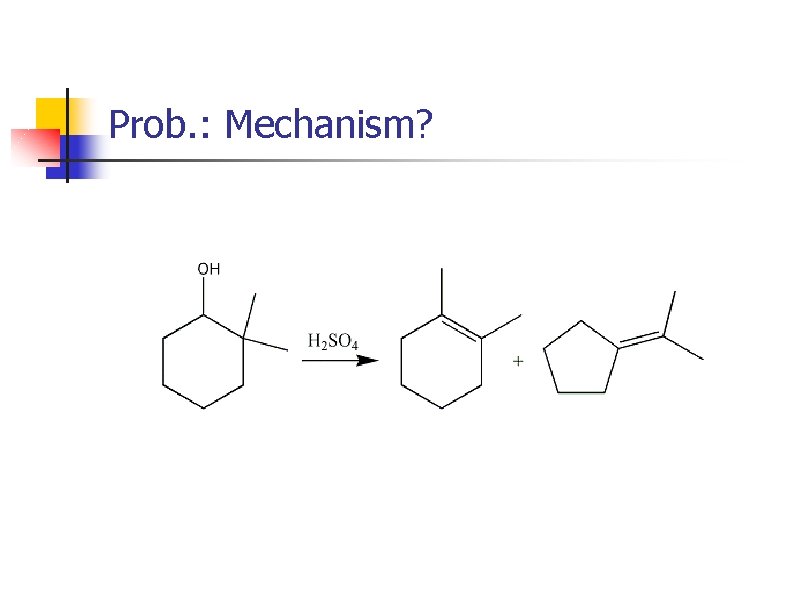
Prob. : Mechanism?
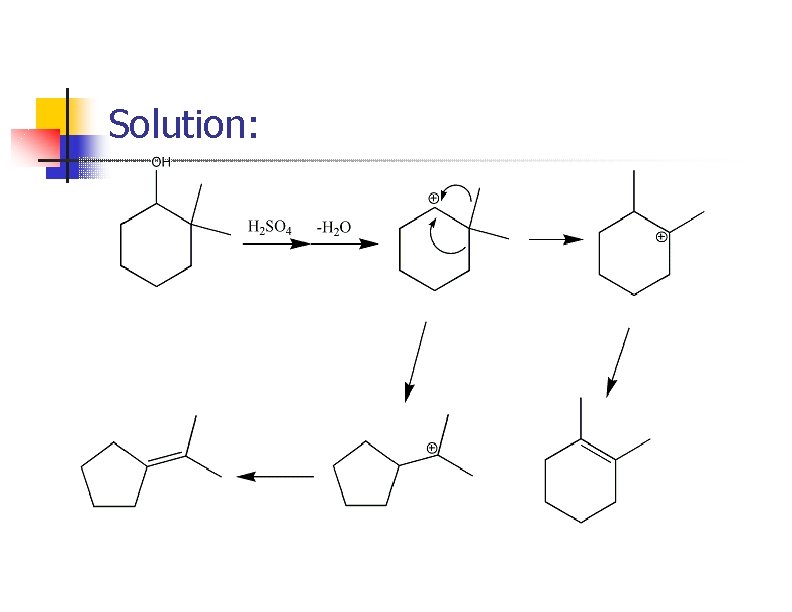
Solution:
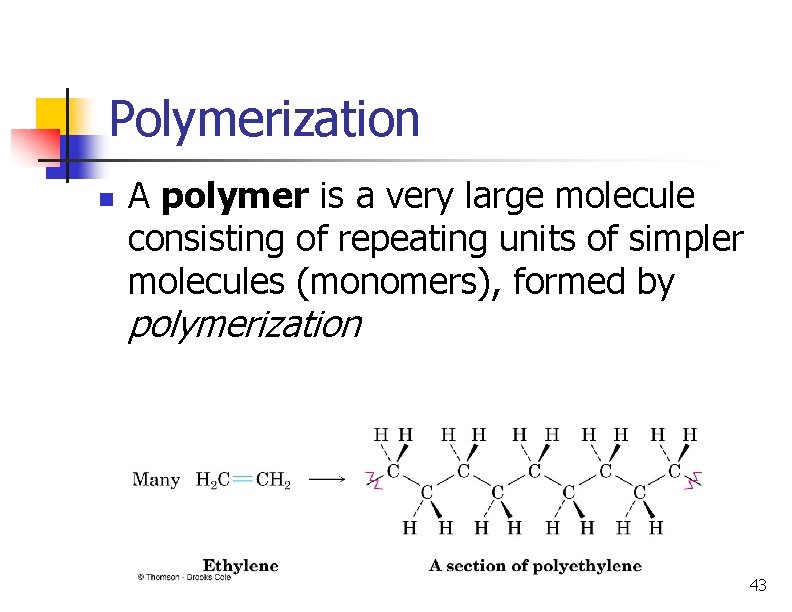
Polymerization n A polymer is a very large molecule consisting of repeating units of simpler molecules (monomers), formed by polymerization 43
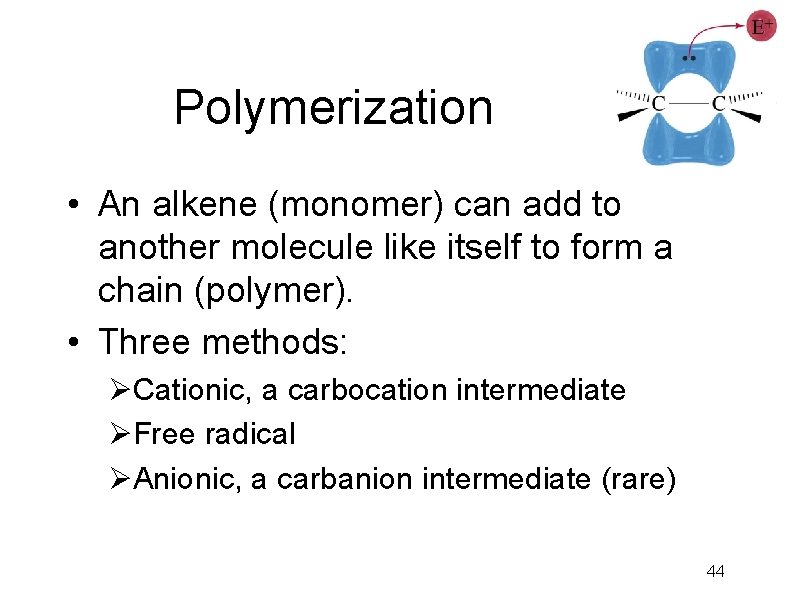
Polymerization • An alkene (monomer) can add to another molecule like itself to form a chain (polymer). • Three methods: ØCationic, a carbocation intermediate ØFree radical ØAnionic, a carbanion intermediate (rare) 44
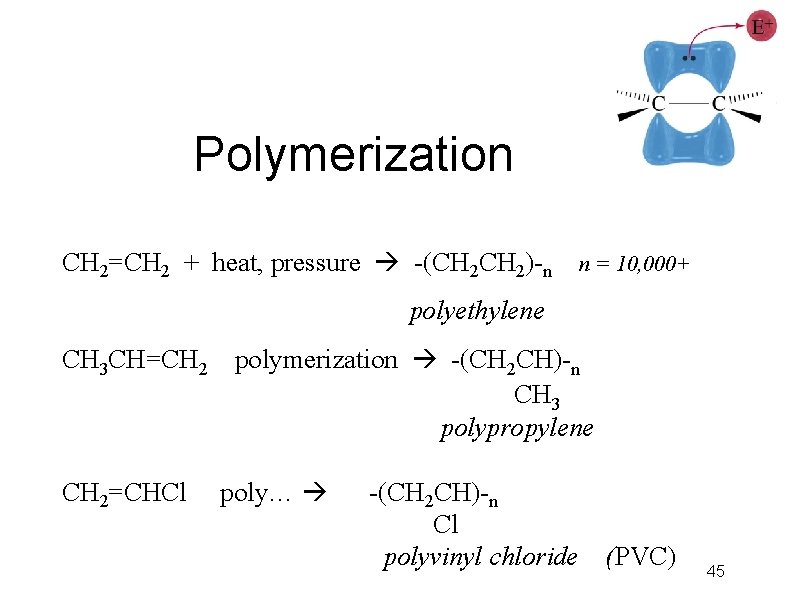
Polymerization CH 2=CH 2 + heat, pressure -(CH 2)-n n = 10, 000+ polyethylene CH 3 CH=CH 2=CHCl polymerization -(CH 2 CH)-n CH 3 polypropylene poly… -(CH 2 CH)-n Cl polyvinyl chloride (PVC) 45
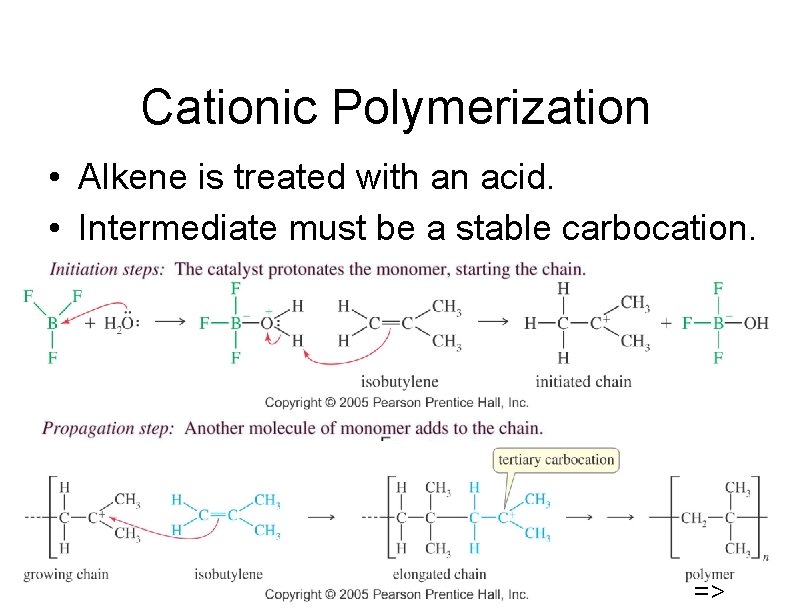
Cationic Polymerization • Alkene is treated with an acid. • Intermediate must be a stable carbocation. 46 =>
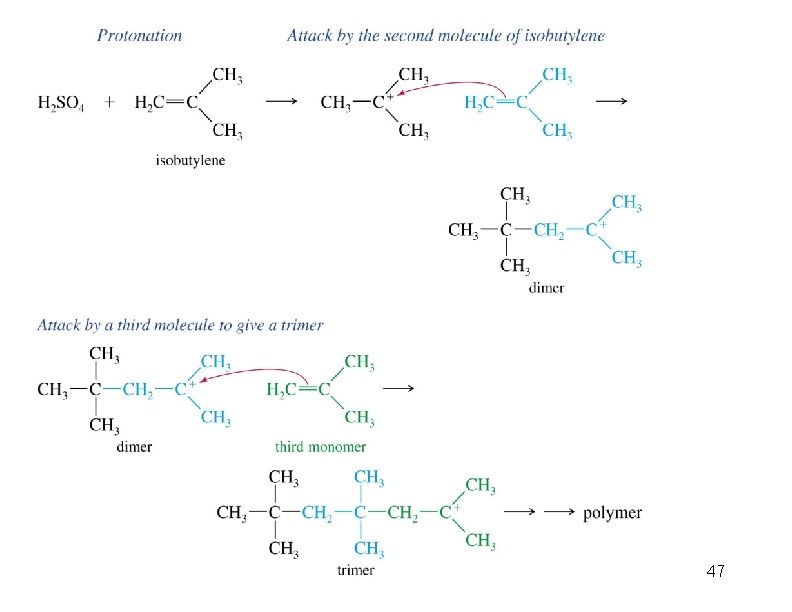
47
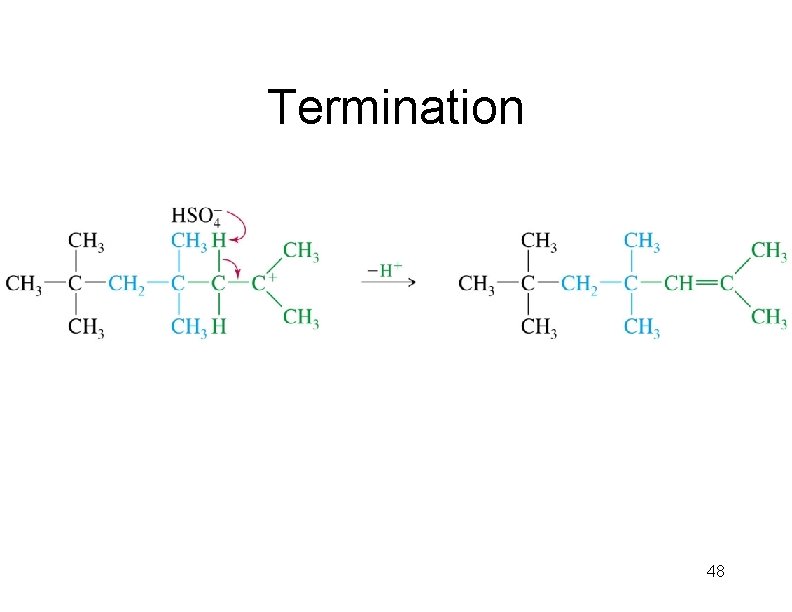
Termination 48
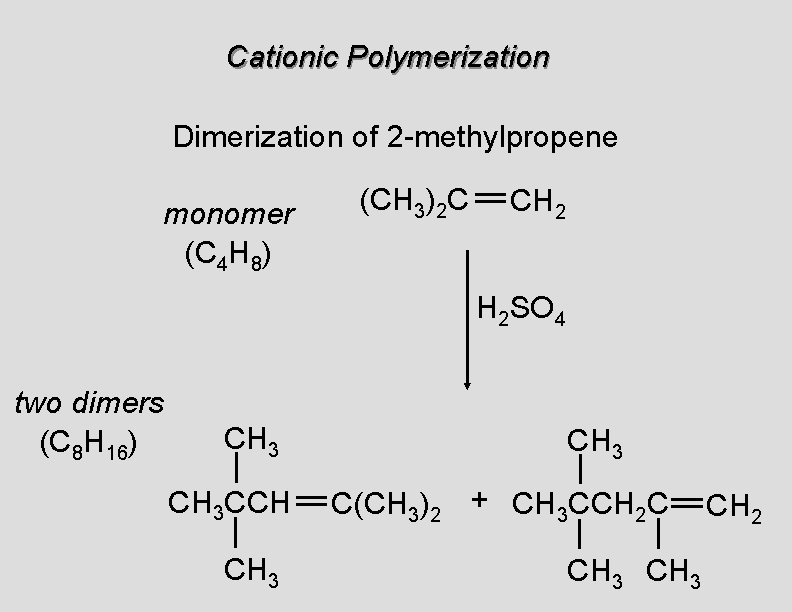
Cationic Polymerization Dimerization of 2 -methylpropene monomer (C 4 H 8) (CH 3)2 C CH 2 H 2 SO 4 two dimers (C 8 H 16) CH 3 CCH CH 3 C(CH 3)2 + CH 3 CCH 2 C CH 3 CH 2
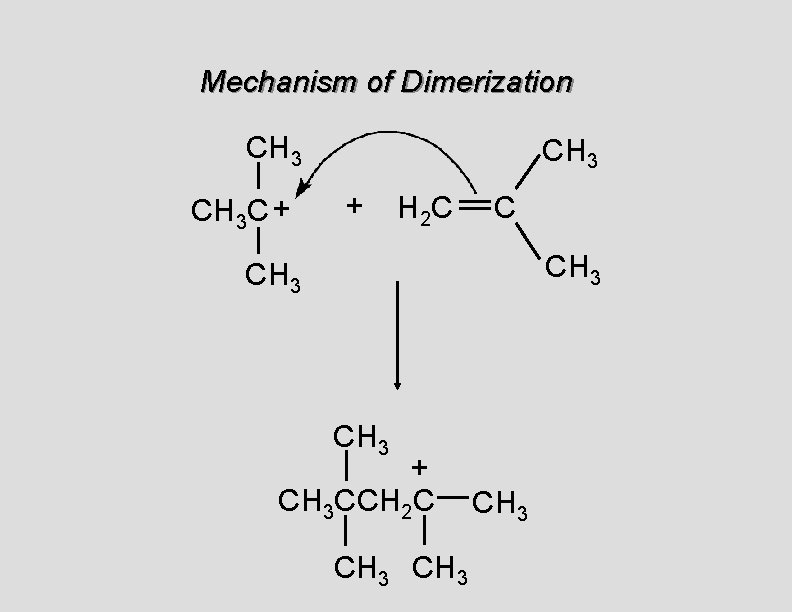
Mechanism of Dimerization CH 3 C + CH 3 + H 2 C C CH 3 + CH 3 CCH 2 C CH 3
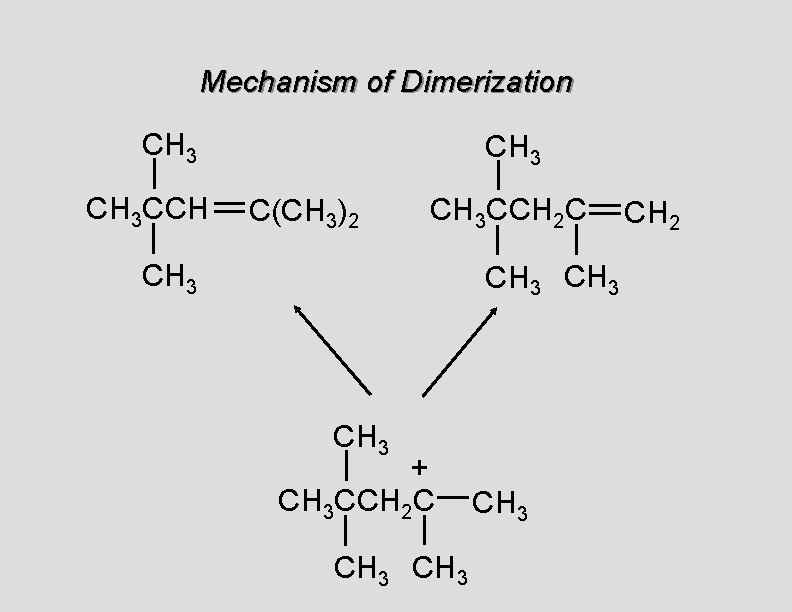
Mechanism of Dimerization CH 3 CCH CH 3 C(CH 3)2 CH 3 CCH 2 C CH 3 + CH 3 CCH 2 C CH 3 CH 2
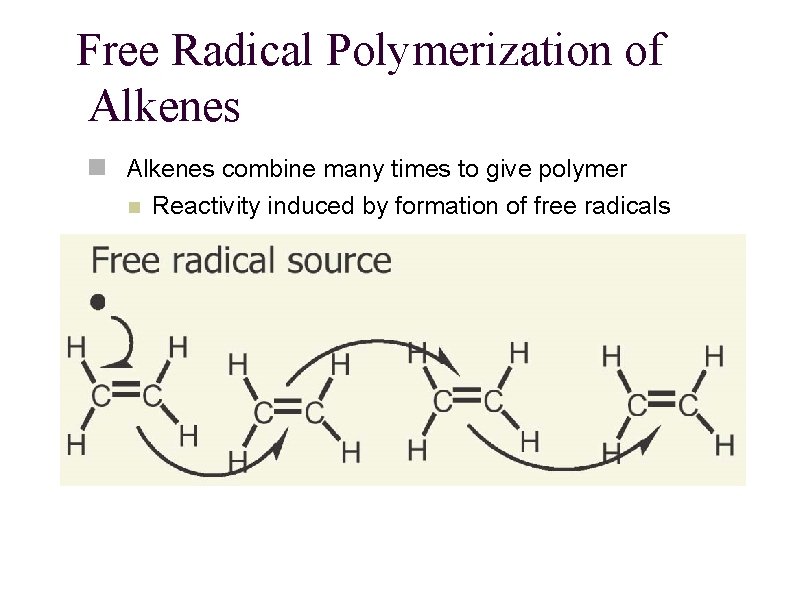
Free Radical Polymerization of Alkenes n Alkenes combine many times to give polymer n Reactivity induced by formation of free radicals
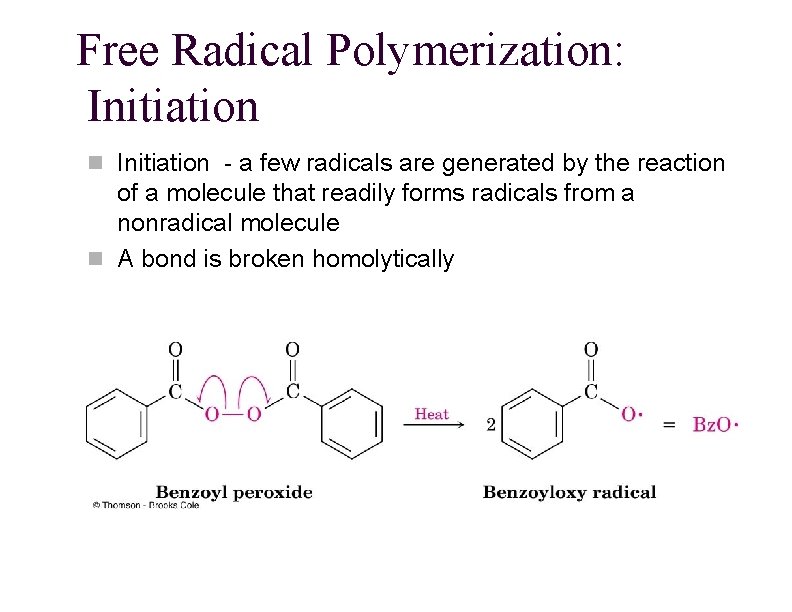
Free Radical Polymerization: Initiation n Initiation - a few radicals are generated by the reaction of a molecule that readily forms radicals from a nonradical molecule n A bond is broken homolytically
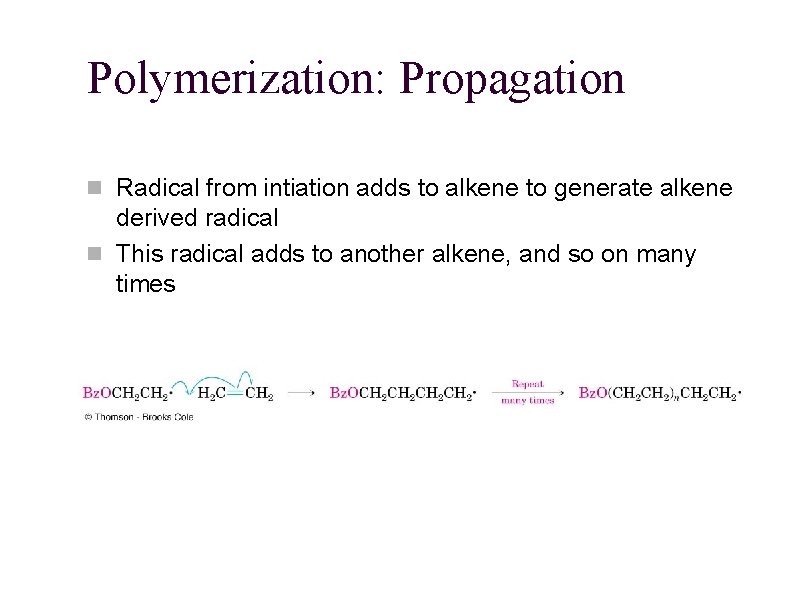
Polymerization: Propagation n Radical from intiation adds to alkene to generate alkene derived radical n This radical adds to another alkene, and so on many times
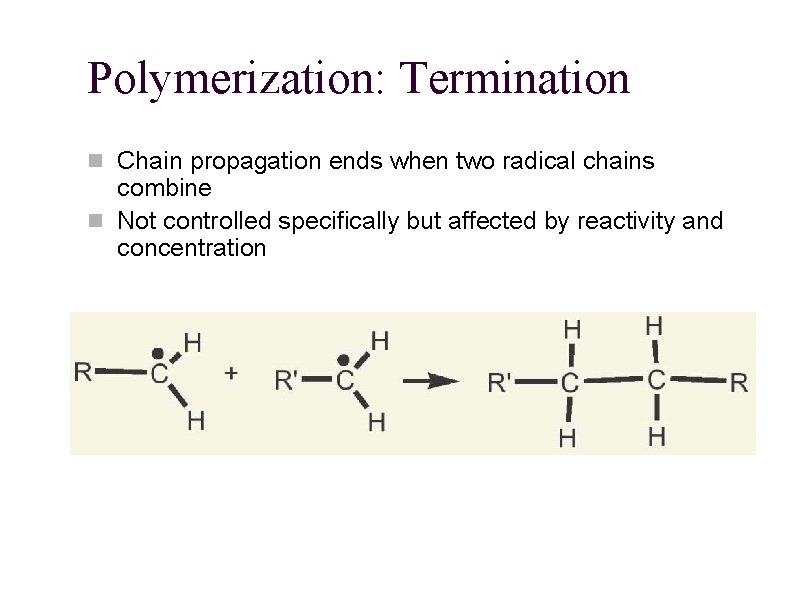
Polymerization: Termination n Chain propagation ends when two radical chains combine n Not controlled specifically but affected by reactivity and concentration
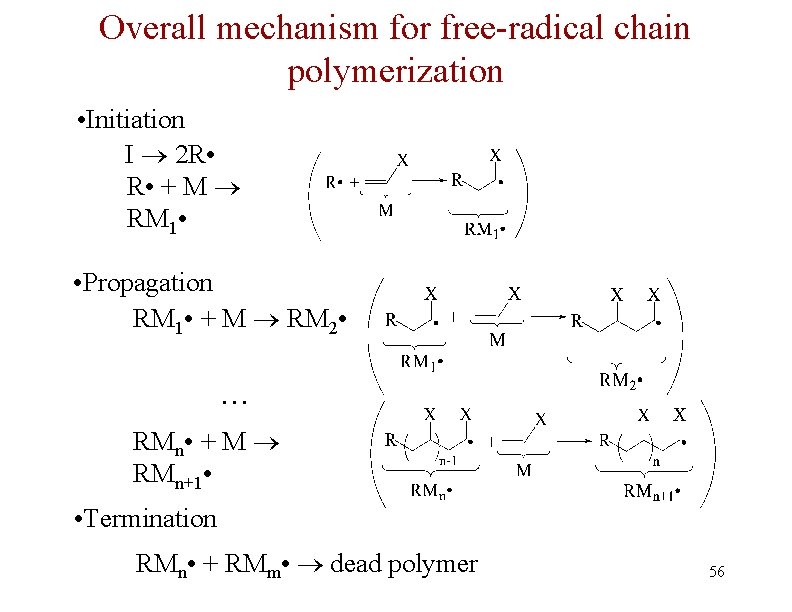
Overall mechanism for free-radical chain polymerization • Initiation I 2 R • + M RM 1 • • Propagation RM 1 • + M RM 2 • … RMn • + M RMn+1 • • Termination RMn • + RMm • dead polymer 56
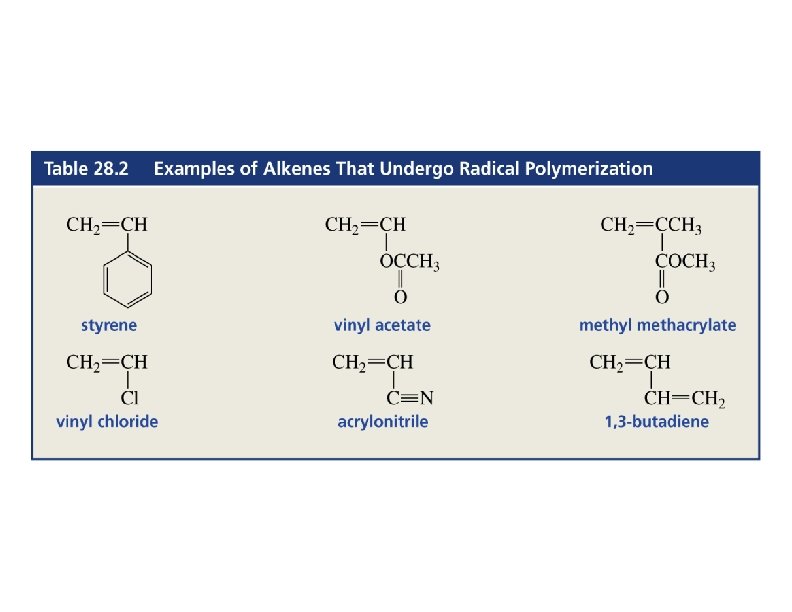
57
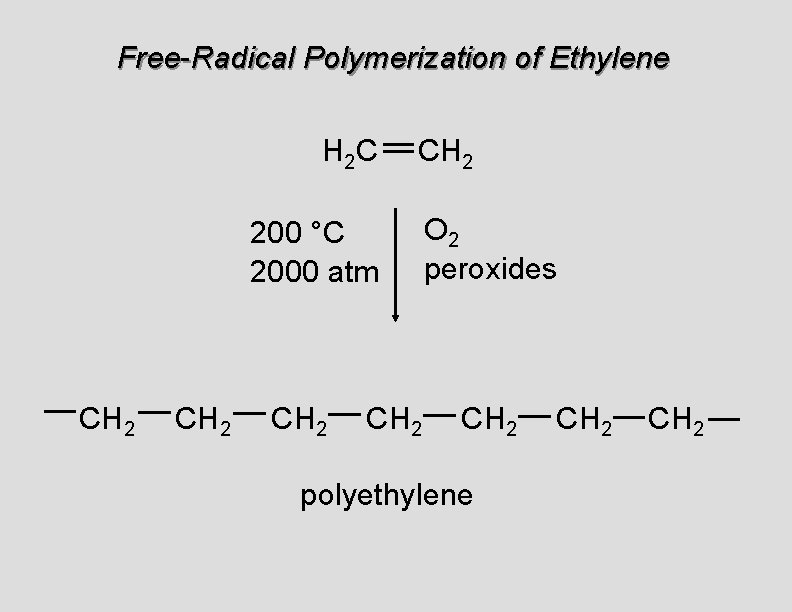
Free-Radical Polymerization of Ethylene H 2 C CH 2 200 °C 2000 atm CH 2 O 2 peroxides CH 2 polyethylene CH 2
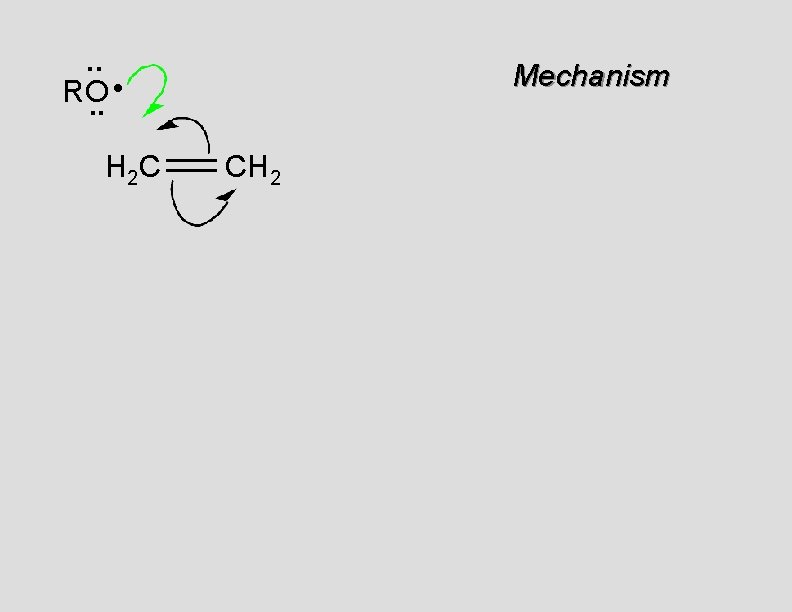
. . • RO. . H 2 C Mechanism CH 2
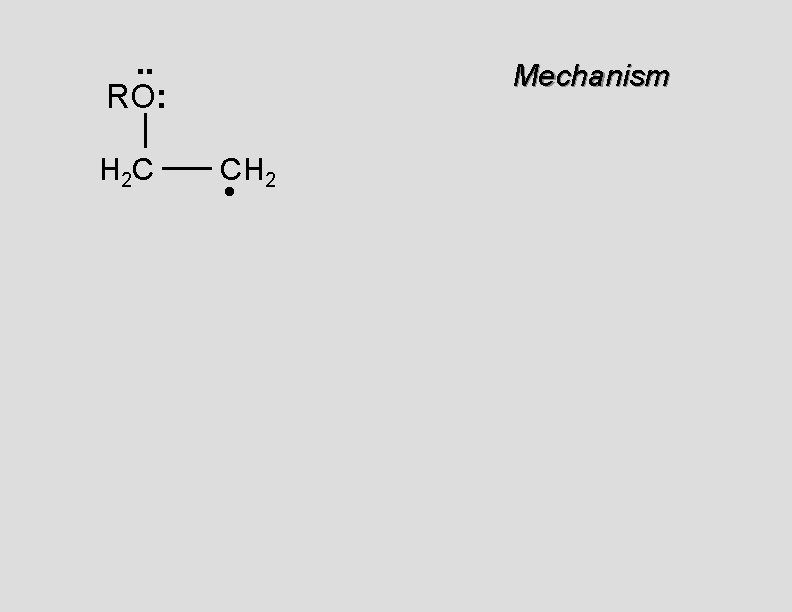
. . RO: H 2 C Mechanism CH 2 •
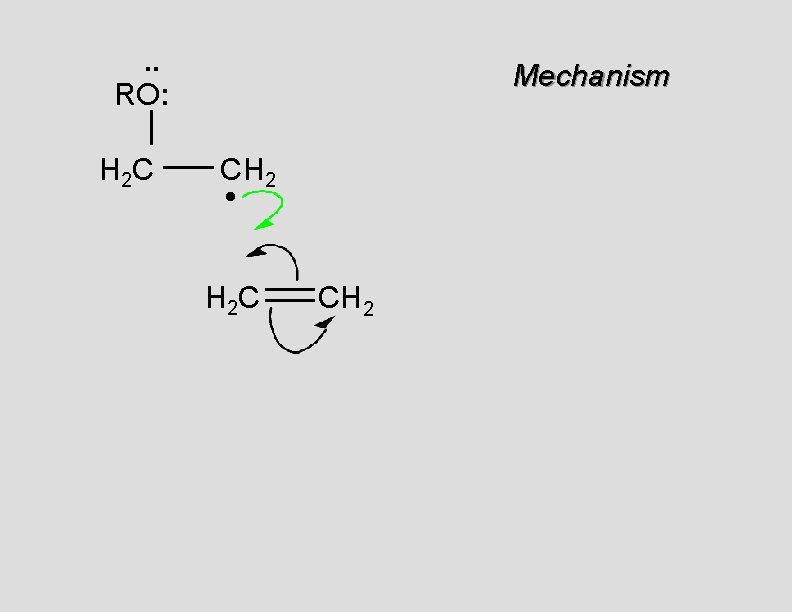
. . RO: H 2 C Mechanism CH 2 • H 2 C CH 2
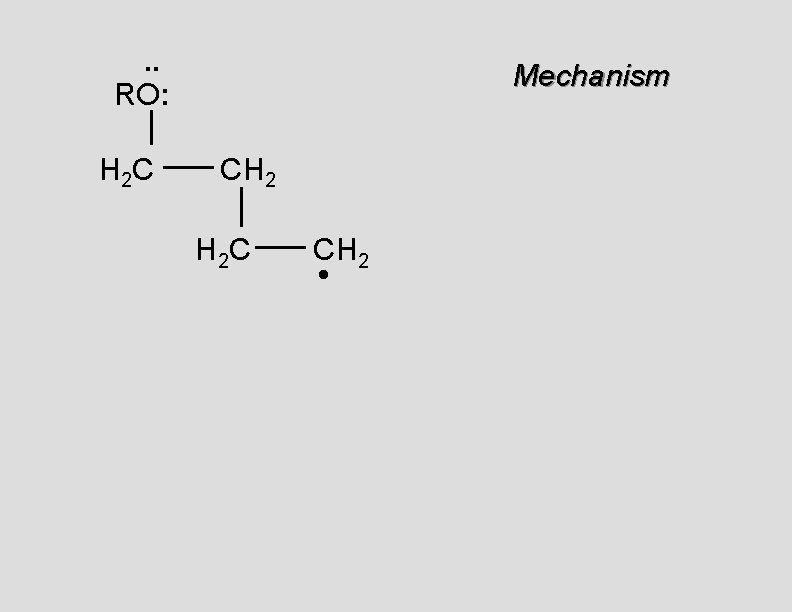
. . RO: H 2 C Mechanism CH 2 H 2 C CH 2 •
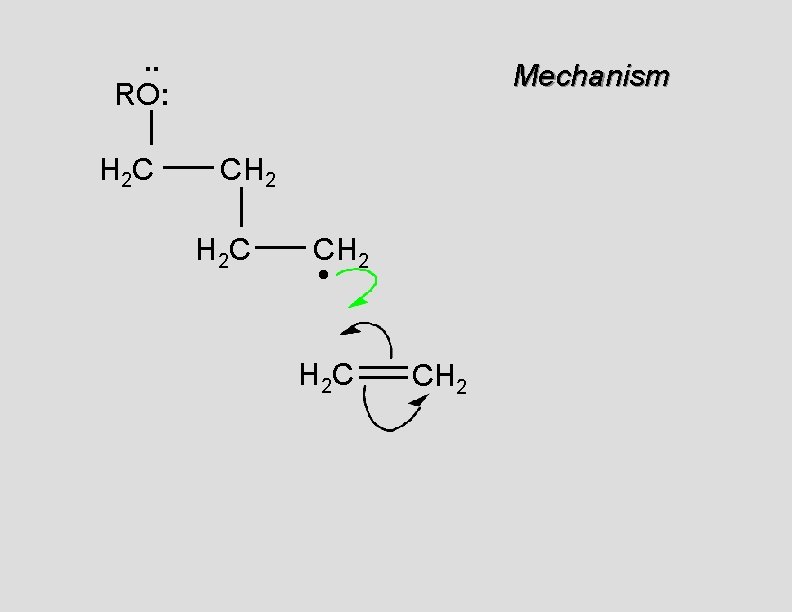
. . RO: H 2 C Mechanism CH 2 H 2 C CH 2 • H 2 C CH 2
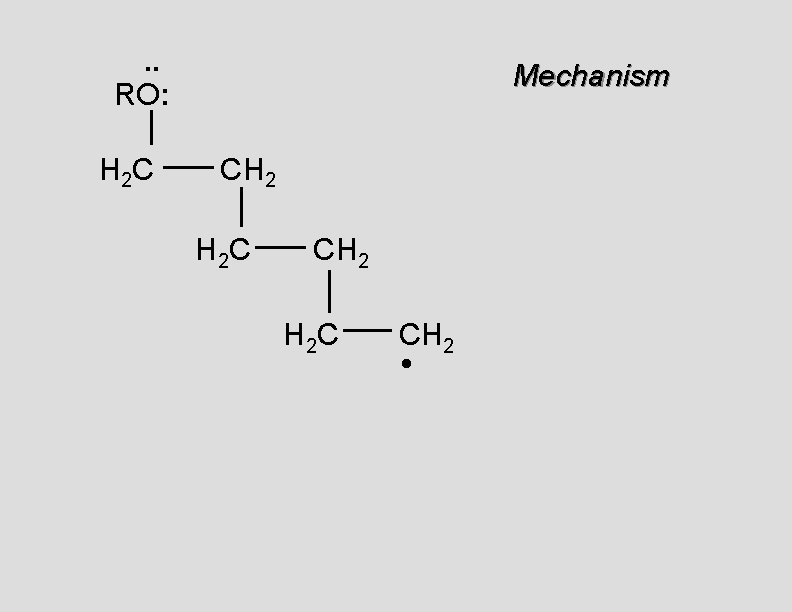
. . RO: H 2 C Mechanism CH 2 H 2 C CH 2 •
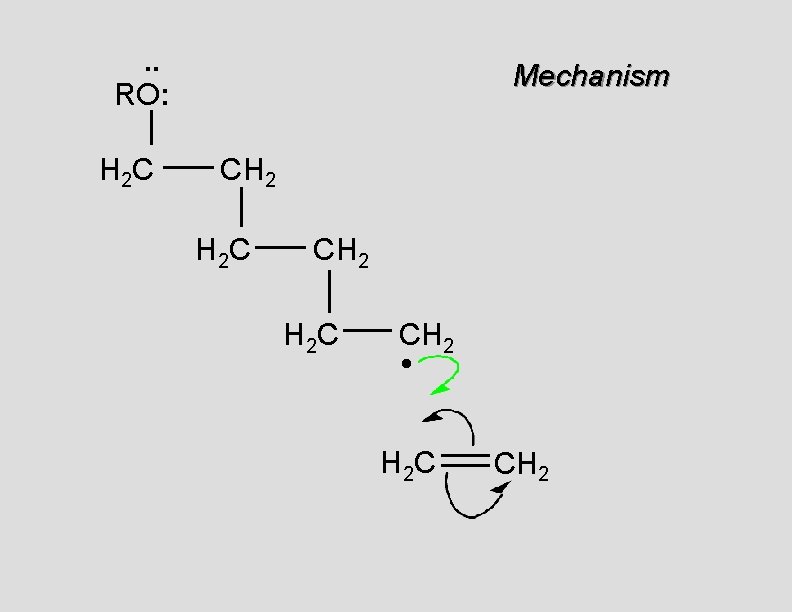
. . RO: H 2 C Mechanism CH 2 H 2 C CH 2 • H 2 C CH 2
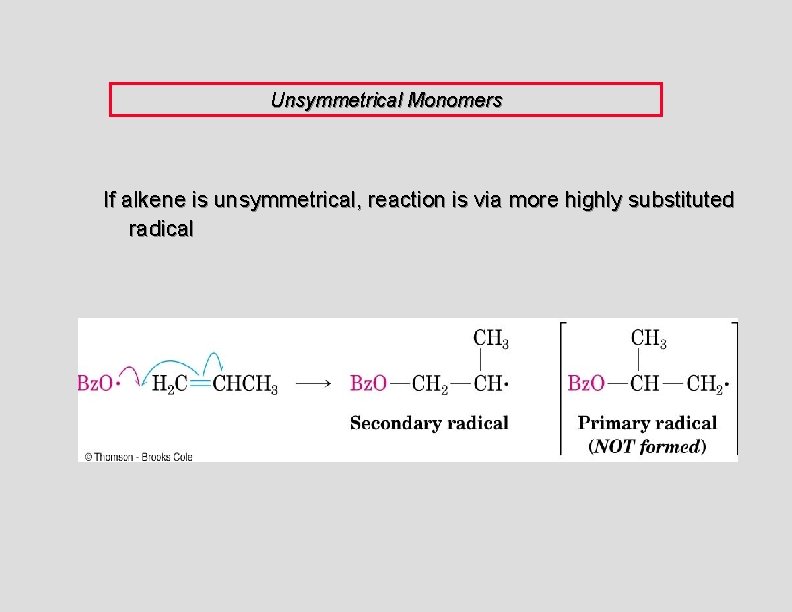
Unsymmetrical Monomers If alkene is unsymmetrical, reaction is via more highly substituted radical
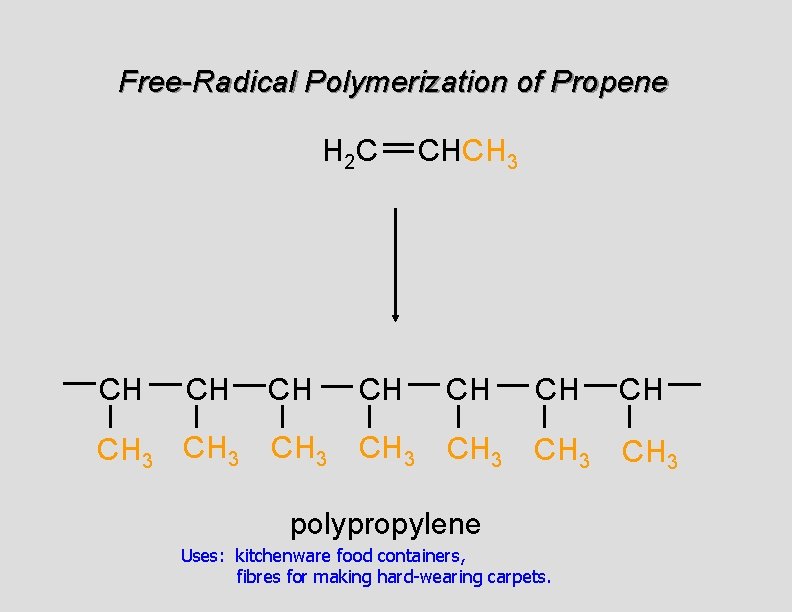
Free-Radical Polymerization of Propene H 2 C CH CH CH 3 CHCH 3 CH CH CH 3 CH 3 polypropylene Uses: kitchenware food containers, fibres for making hard-wearing carpets.
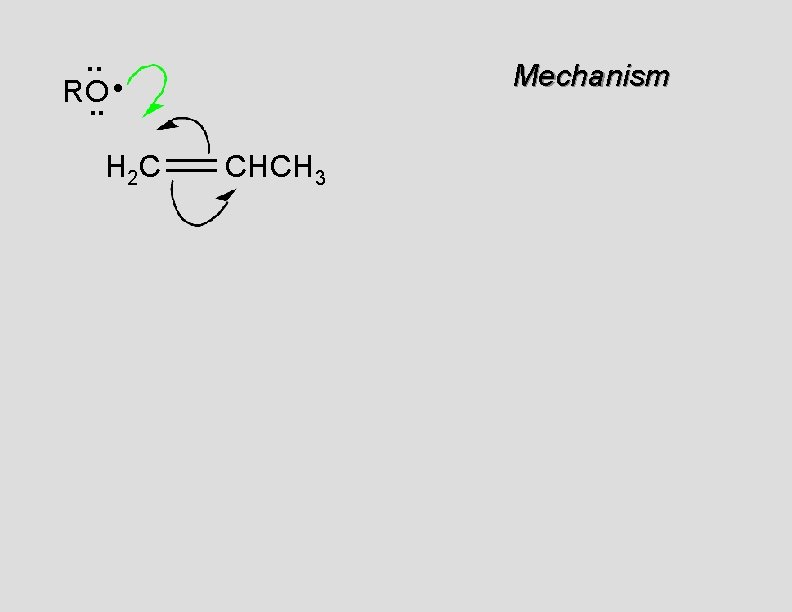
. . • RO. . H 2 C Mechanism CHCH 3
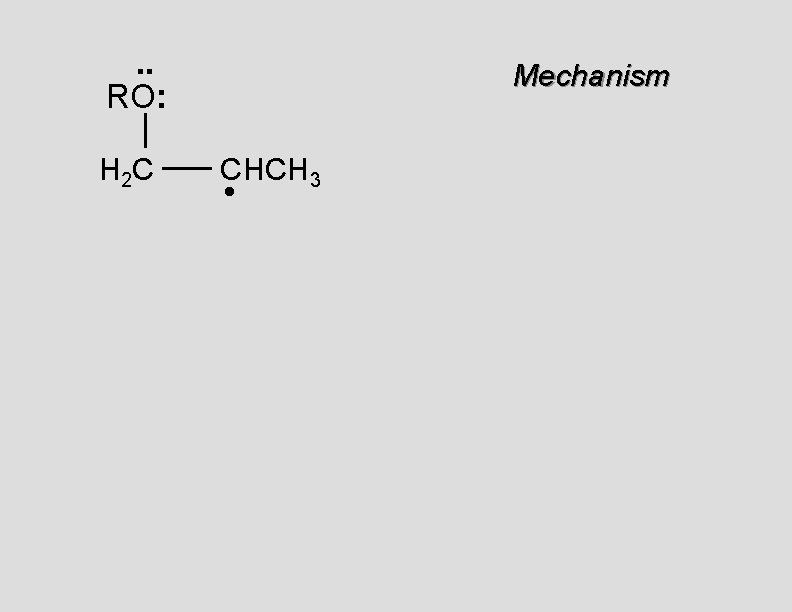
. . RO: H 2 C Mechanism CHCH 3 •
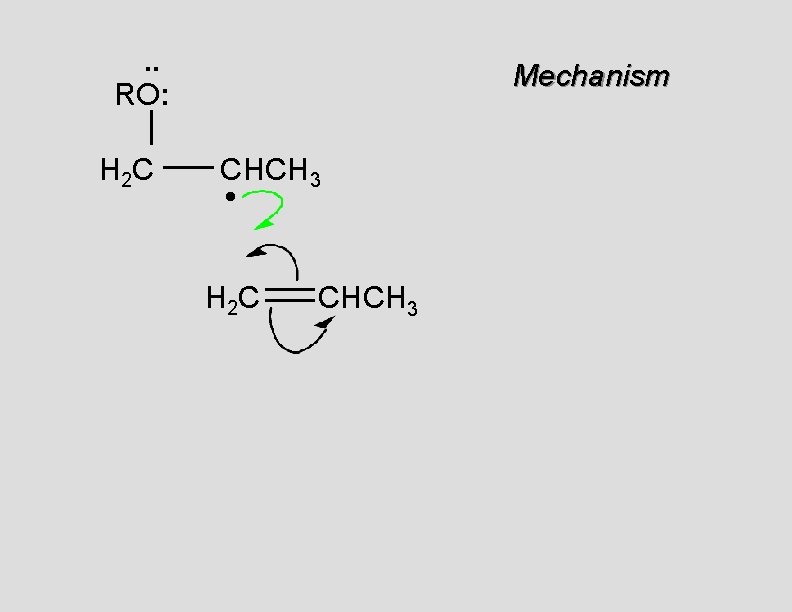
. . RO: H 2 C Mechanism CHCH 3 • H 2 C CHCH 3
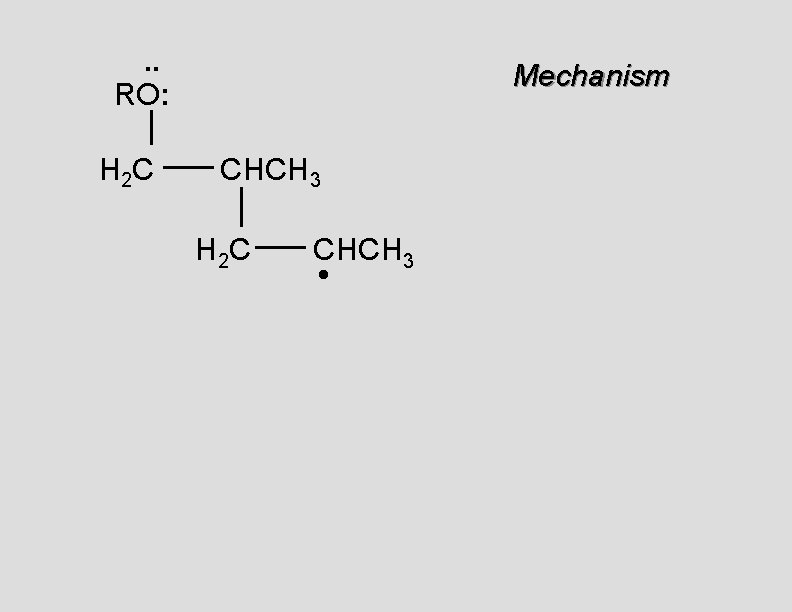
. . RO: H 2 C Mechanism CHCH 3 H 2 C CHCH 3 •
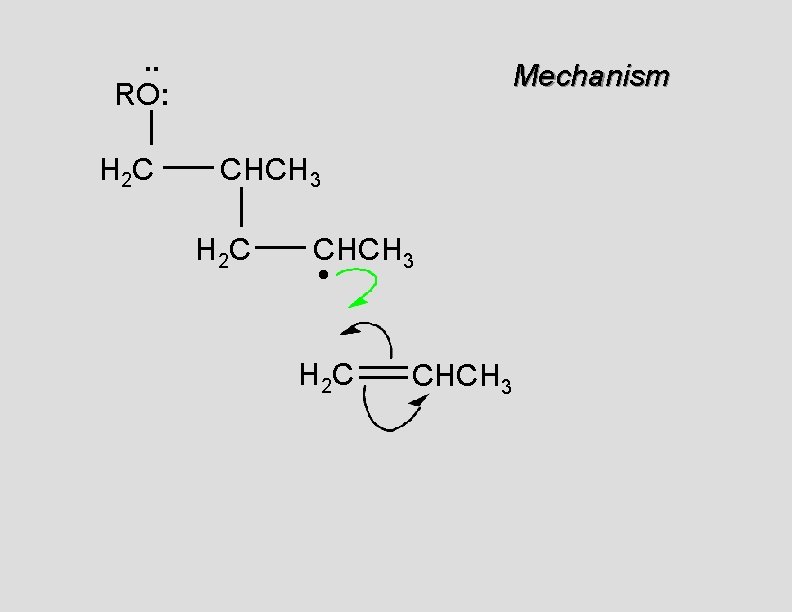
. . RO: H 2 C Mechanism CHCH 3 H 2 C CHCH 3 • H 2 C CHCH 3
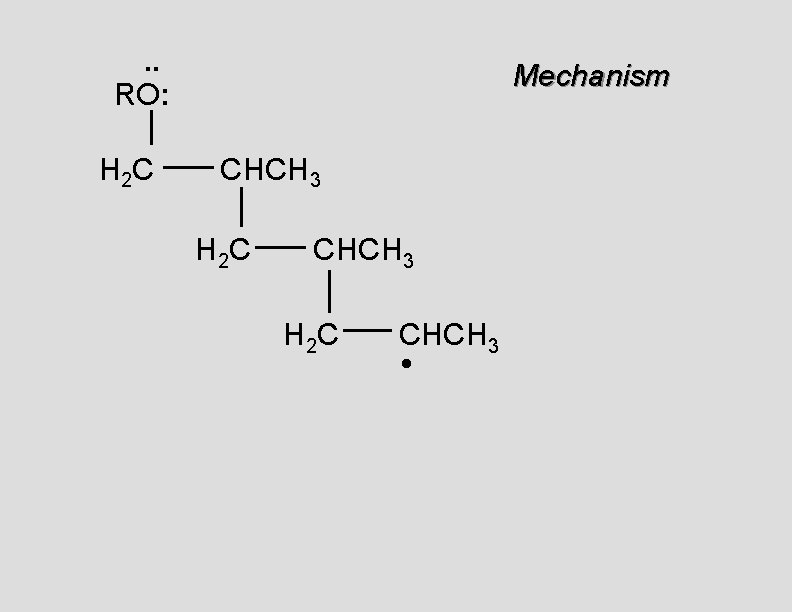
. . RO: H 2 C Mechanism CHCH 3 H 2 C CHCH 3 •
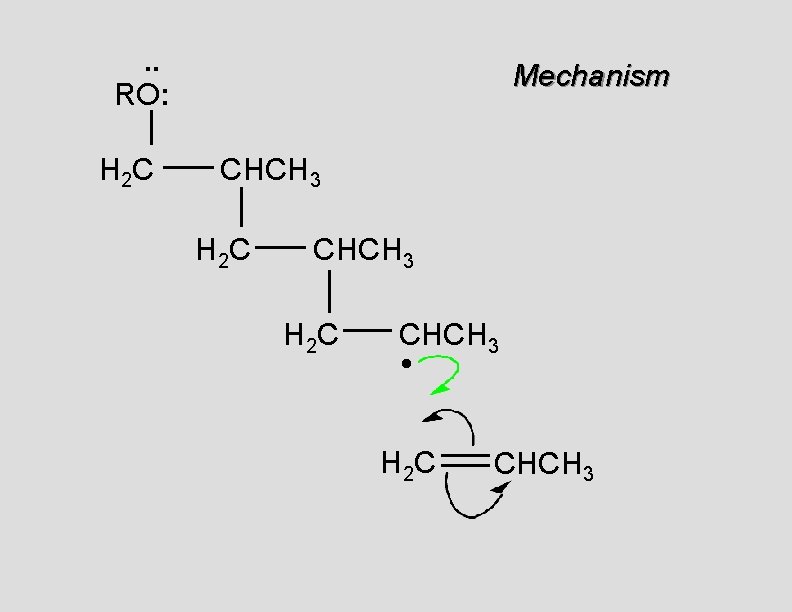
. . RO: H 2 C Mechanism CHCH 3 H 2 C CHCH 3 • H 2 C CHCH 3
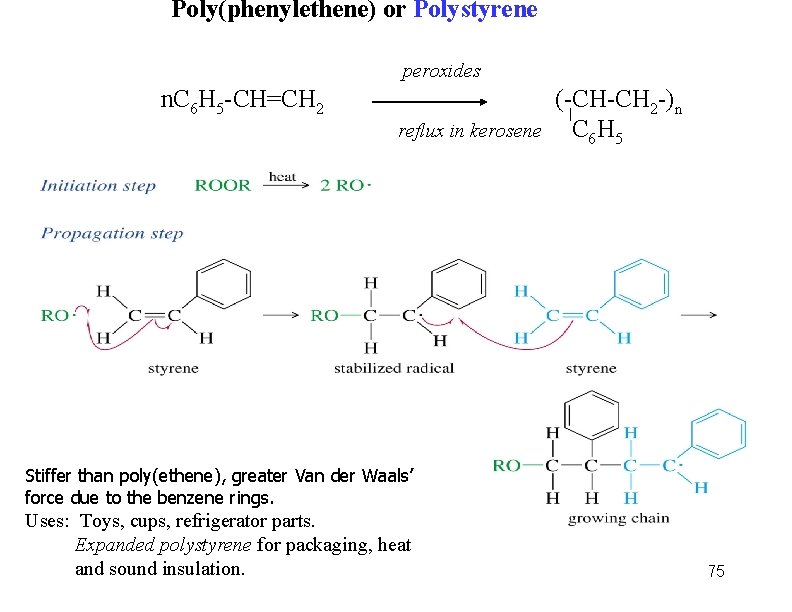
Poly(phenylethene) or Polystyrene peroxides n. C 6 H 5 -CH=CH 2 reflux in kerosene (-CH-CH 2 -)n C 6 H 5 Stiffer than poly(ethene), greater Van der Waals’ force due to the benzene rings. Uses: Toys, cups, refrigerator parts. Expanded polystyrene for packaging, heat and sound insulation. 75
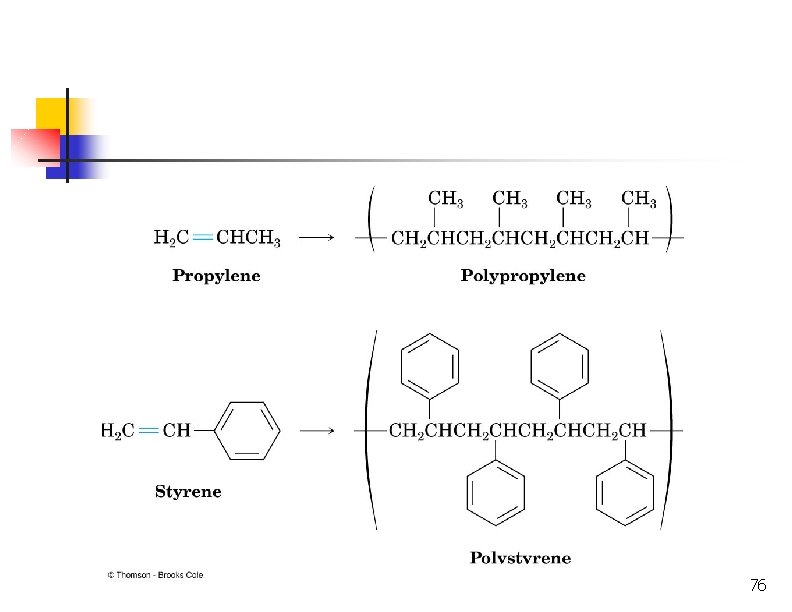
76
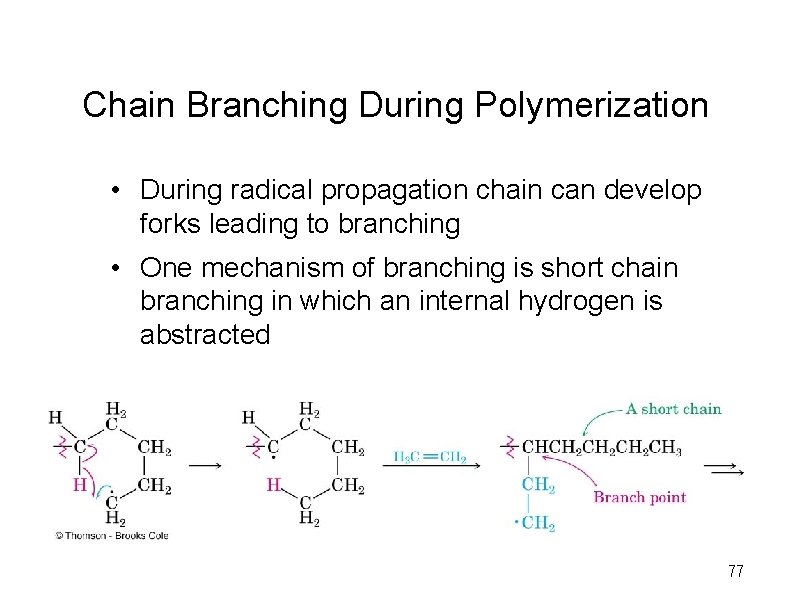
Chain Branching During Polymerization • During radical propagation chain can develop forks leading to branching • One mechanism of branching is short chain branching in which an internal hydrogen is abstracted 77
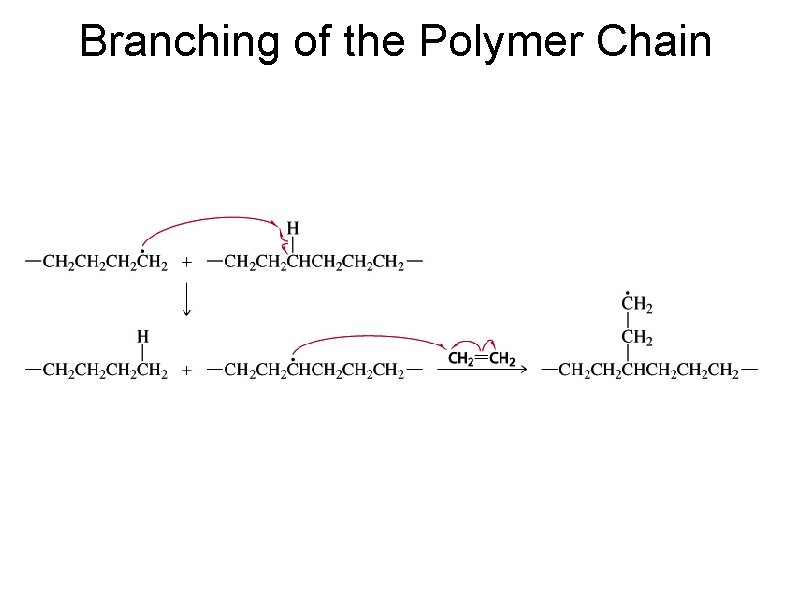
Branching of the Polymer Chain 78
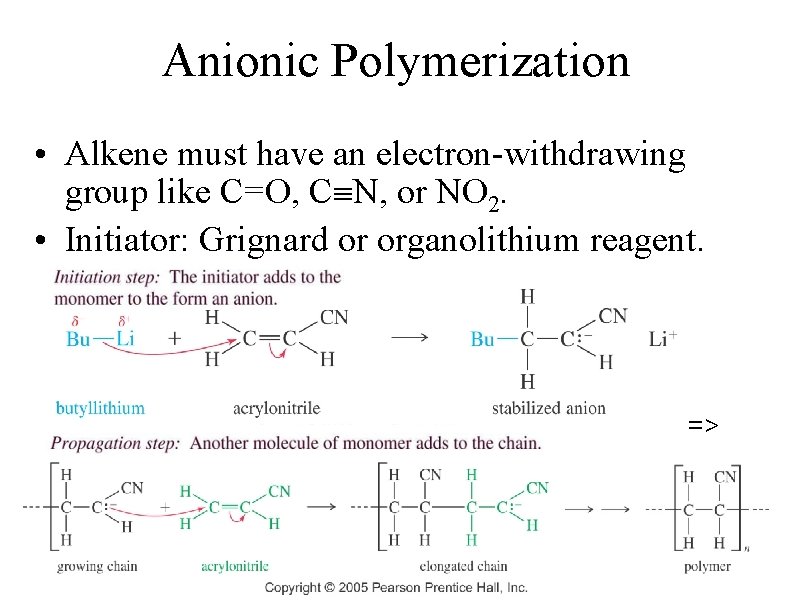
Anionic Polymerization • Alkene must have an electron-withdrawing group like C=O, C N, or NO 2. • Initiator: Grignard or organolithium reagent. => 79
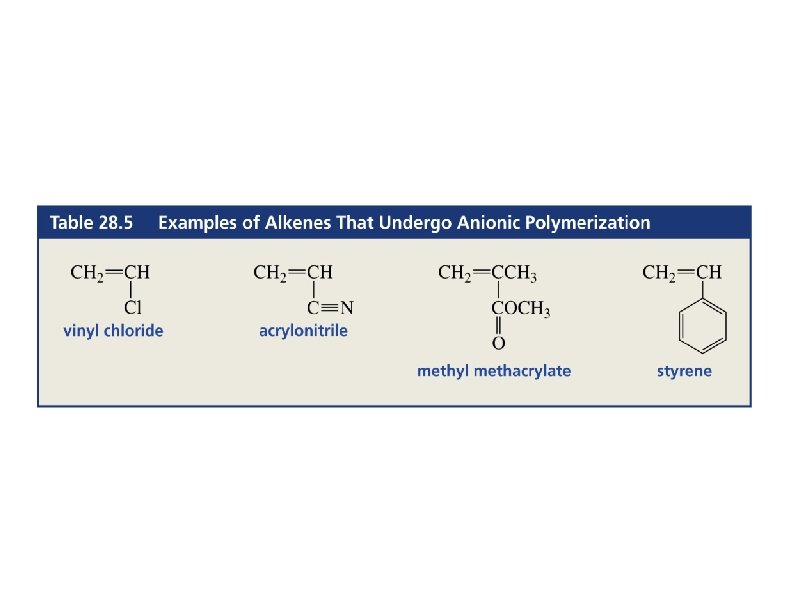
80
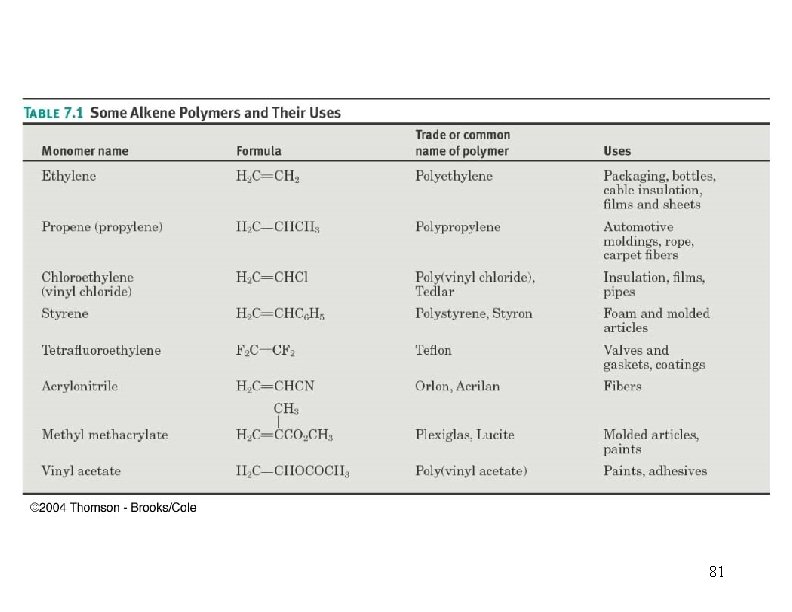
81
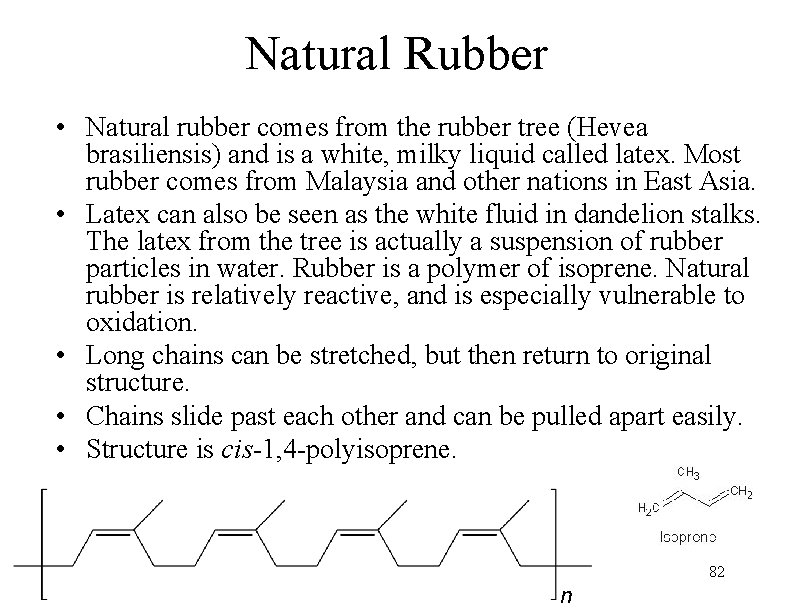
Natural Rubber • Natural rubber comes from the rubber tree (Hevea brasiliensis) and is a white, milky liquid called latex. Most rubber comes from Malaysia and other nations in East Asia. • Latex can also be seen as the white fluid in dandelion stalks. The latex from the tree is actually a suspension of rubber particles in water. Rubber is a polymer of isoprene. Natural rubber is relatively reactive, and is especially vulnerable to oxidation. • Long chains can be stretched, but then return to original structure. • Chains slide past each other and can be pulled apart easily. • Structure is cis-1, 4 -polyisoprene. 82 n
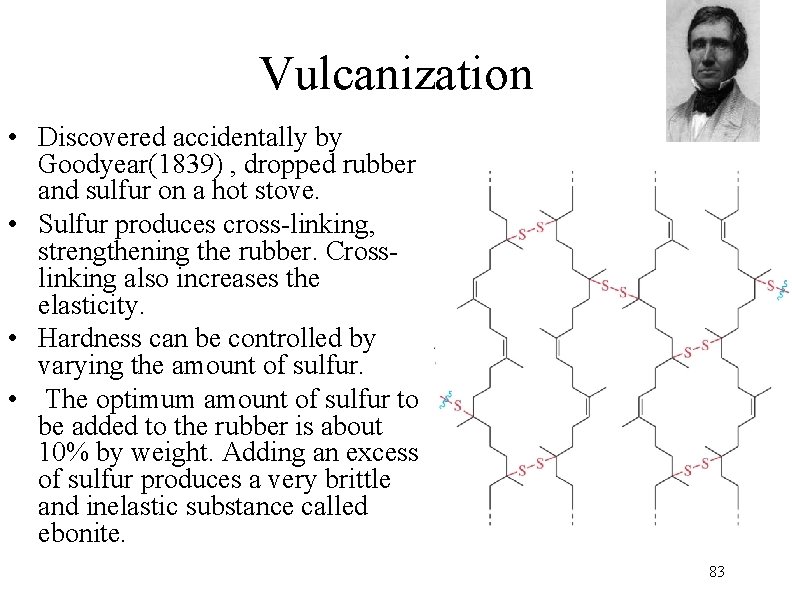
Vulcanization • Discovered accidentally by Goodyear(1839) , dropped rubber and sulfur on a hot stove. • Sulfur produces cross-linking, strengthening the rubber. Crosslinking also increases the elasticity. • Hardness can be controlled by varying the amount of sulfur. • The optimum amount of sulfur to be added to the rubber is about 10% by weight. Adding an excess of sulfur produces a very brittle and inelastic substance called ebonite. 83
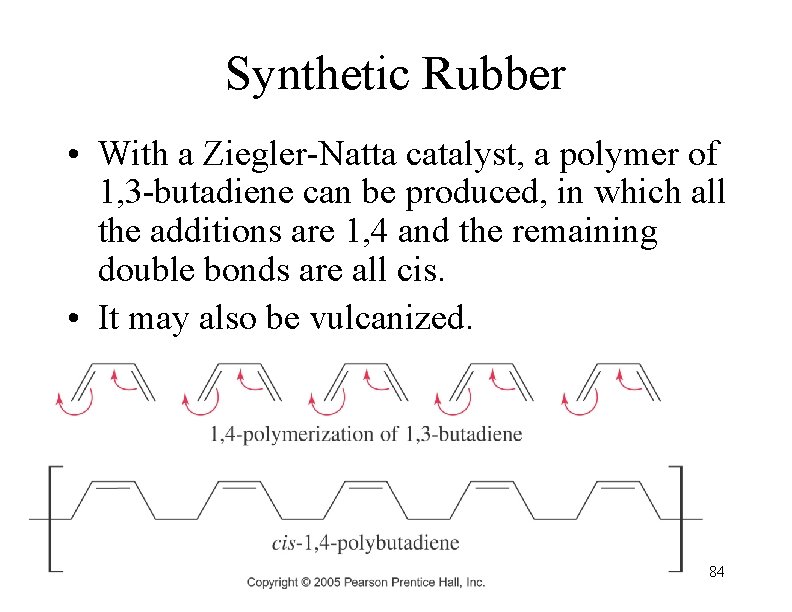
Synthetic Rubber • With a Ziegler-Natta catalyst, a polymer of 1, 3 -butadiene can be produced, in which all the additions are 1, 4 and the remaining double bonds are all cis. • It may also be vulcanized. 84
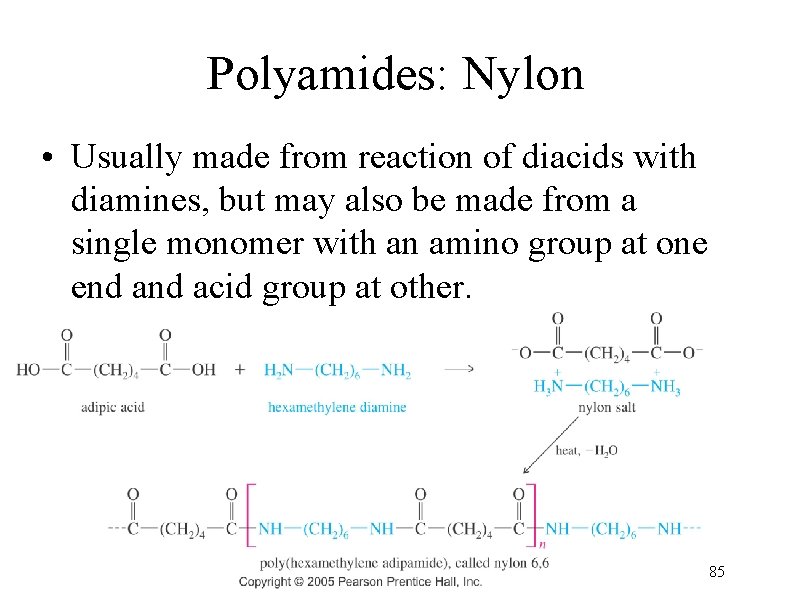
Polyamides: Nylon • Usually made from reaction of diacids with diamines, but may also be made from a single monomer with an amino group at one end acid group at other. 85
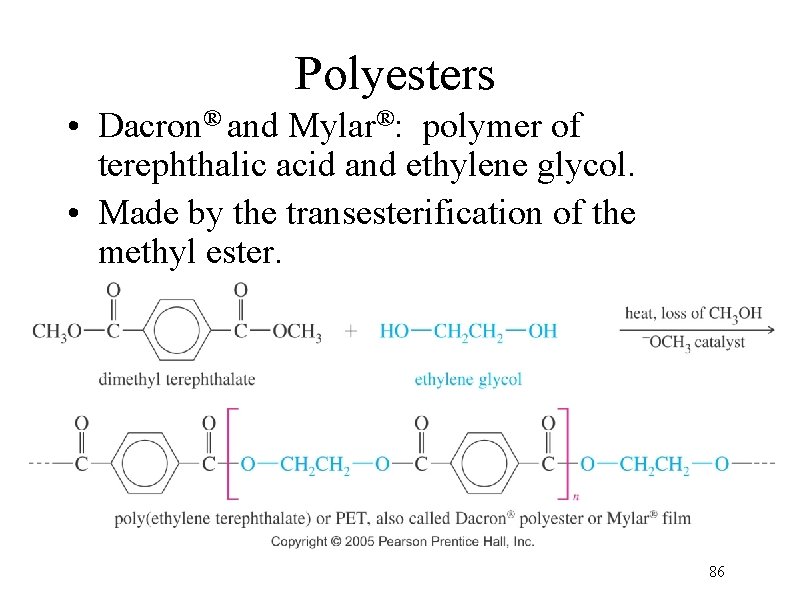
Polyesters • Dacron® and Mylar®: polymer of terephthalic acid and ethylene glycol. • Made by the transesterification of the methyl ester. 86
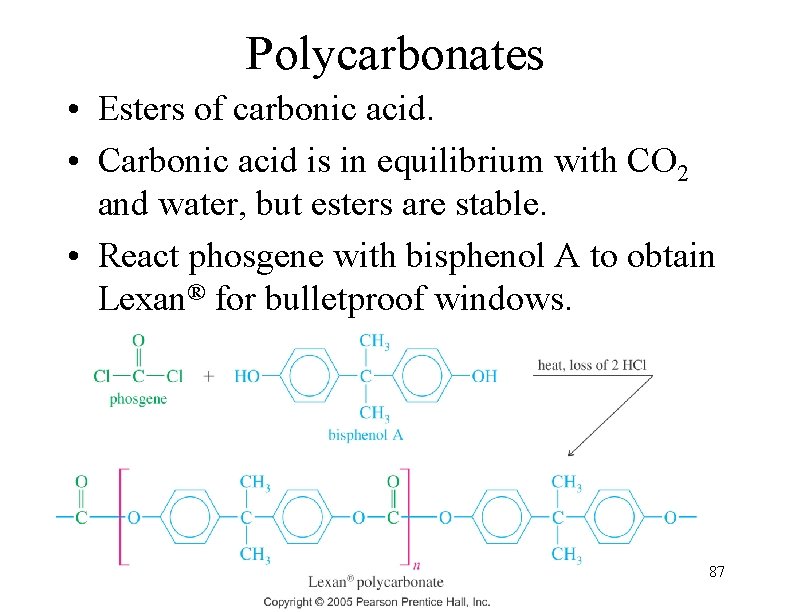
Polycarbonates • Esters of carbonic acid. • Carbonic acid is in equilibrium with CO 2 and water, but esters are stable. • React phosgene with bisphenol A to obtain Lexan® for bulletproof windows. 87
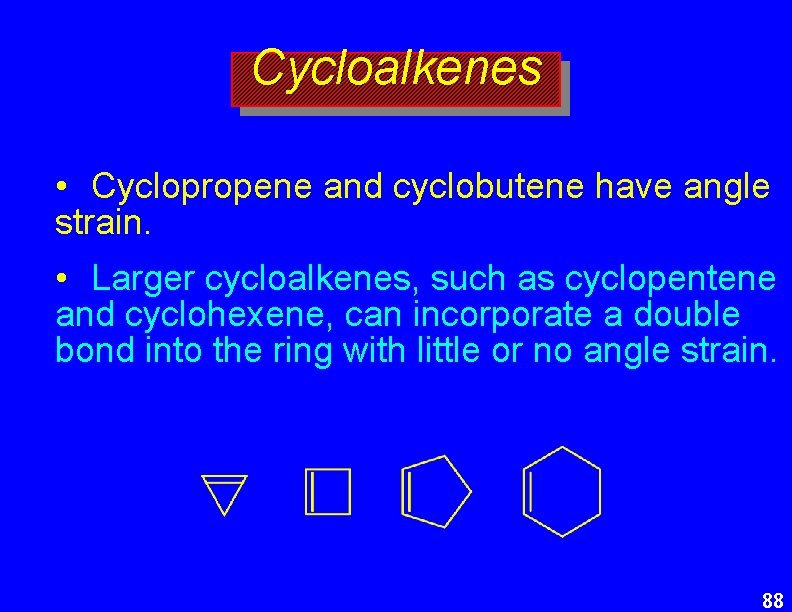
Cycloalkenes • Cyclopropene and cyclobutene have angle strain. • Larger cycloalkenes, such as cyclopentene and cyclohexene, can incorporate a double bond into the ring with little or no angle strain. 88
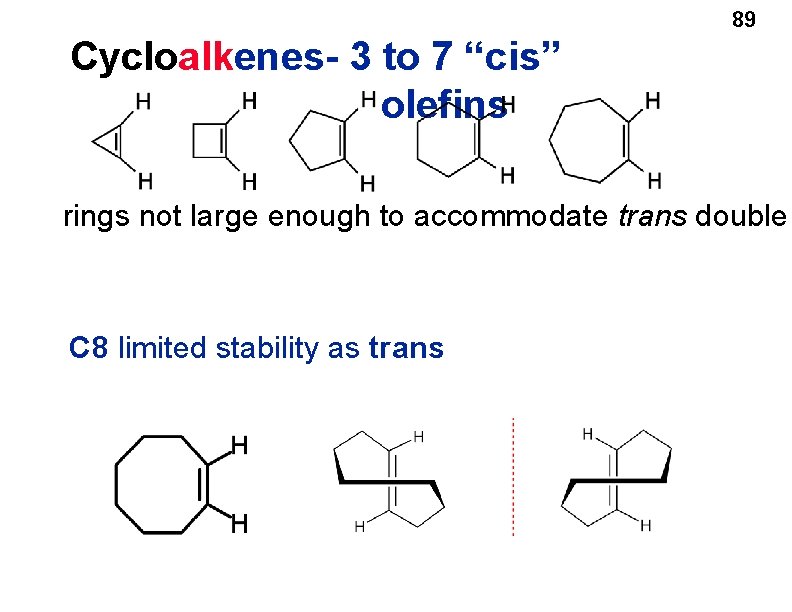
89 Cycloalkenes- 3 to 7 “cis” olefins rings not large enough to accommodate trans double C 8 limited stability as trans
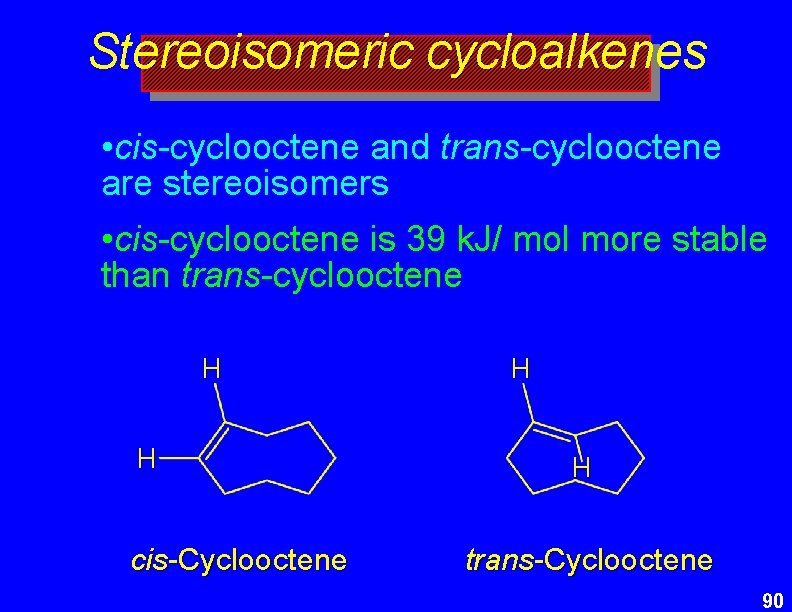
Stereoisomeric cycloalkenes • cis-cyclooctene and trans-cyclooctene are stereoisomers • cis-cyclooctene is 39 k. J/ mol more stable than trans-cyclooctene H H cis-Cyclooctene H H trans-Cyclooctene 90
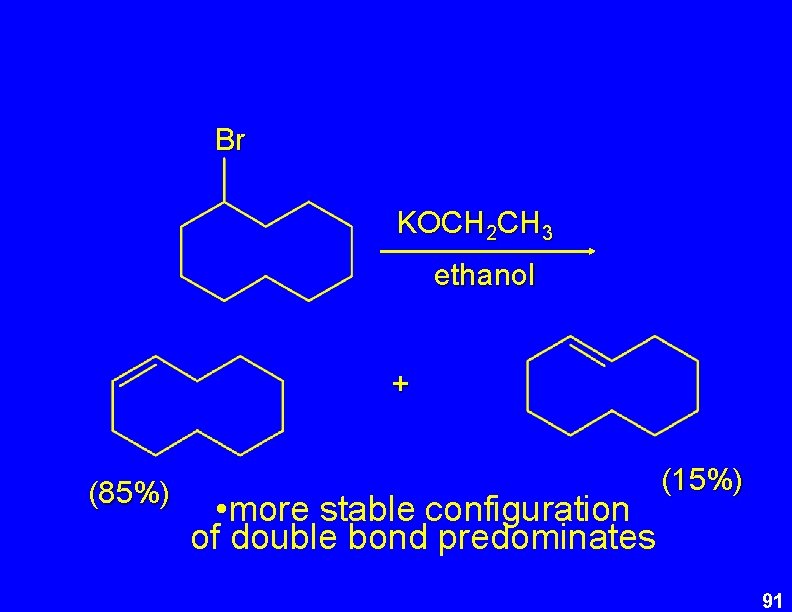
Br KOCH 2 CH 3 ethanol + (85%) • more stable configuration of double bond predominates (15%) 91
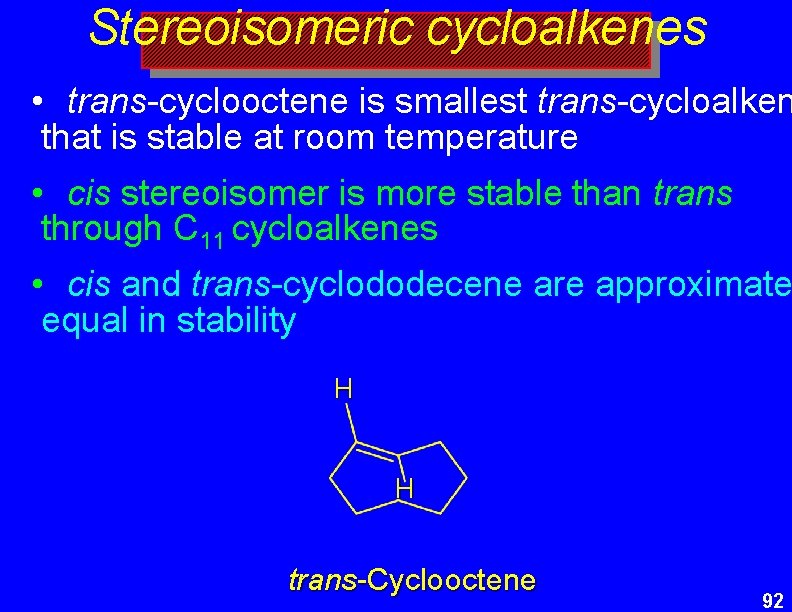
Stereoisomeric cycloalkenes • trans-cyclooctene is smallest trans-cycloalken that is stable at room temperature • cis stereoisomer is more stable than trans through C 11 cycloalkenes • cis and trans-cyclododecene are approximate equal in stability H H trans-Cyclooctene 92
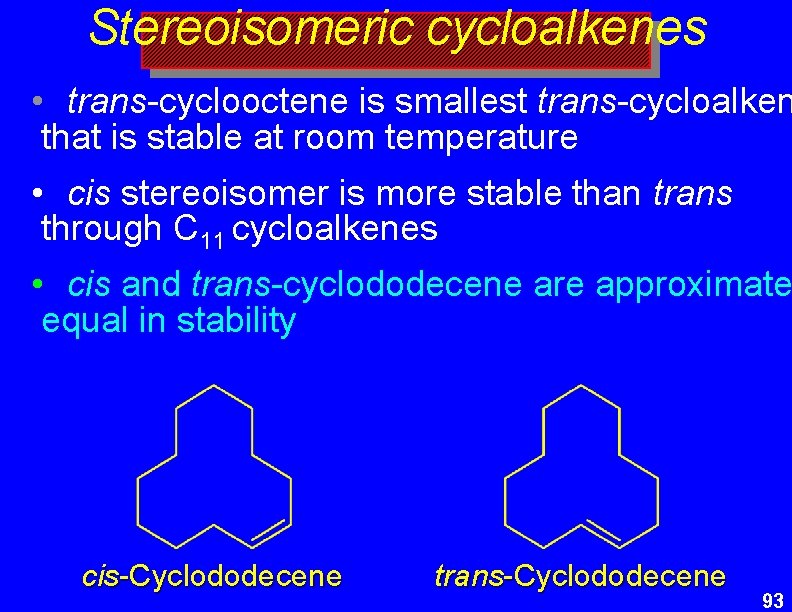
Stereoisomeric cycloalkenes • trans-cyclooctene is smallest trans-cycloalken that is stable at room temperature • cis stereoisomer is more stable than trans through C 11 cycloalkenes • cis and trans-cyclododecene are approximate equal in stability cis-Cyclododecene trans-Cyclododecene 93
Inorganic vs organic chemistry
Ib organic chemistry
Nit calicut chemistry
Hono organic chemistry
Ee organic chemistry
Chapter 22 review organic chemistry section 1 answers
Which allotrope of carbon feels greasy and crumbles easily?
Kiliani fischer synthesis
Organic chemistry
Mindup mind map
Organic chemistry
Analytical chemistry chapters
Ag schnelltest beilstein
What is a branched hydrocarbon
How to calculate percent yield
Is ch4o organic or inorganic
Wiley
Organic chemistry
Is alkane an organic compound
Conjugation organic chemistry
Organic chemistry myanmar
Ester organic chemistry
Grade 10 organic chemistry
Resonance in benzyl carbocation
Functional group
Chemistry ethics case studies
Where is lysine found
A level chemistry ocr organic synthesis
Vicinal dihalide
Ario organic chemistry
Organic chemistry reaction pathways
Halohydrin formation
Pent hex hept oct
Klein organic chemistry 2nd edition
Organic chemistry stuart warren
Brooklyn college organic chemistry
Hammond's postulate organic chemistry
Polarimetry organic chemistry
Hammond's postulate organic chemistry
Organic chemistry nova
What is the leveling effect organic chemistry
Organic chemistry vocabulary
David klein
Organic chemistry
Alkene formula
Organic chemistry
Ethene hbr
Organic chemistry
Cyclo organic chemistry
What functional group is ch3
Organic chemistry
Organic chemistry william h brown
Organic chemistry class 11 notes
Rancidity meaning
Organic chemistry topic 11
Organic chemistry (3rd) edition chapter 1 problem 16s
Alkene alcohol naming
All structural isomers of hexane
Ir spectroscopy
How is cracking done
Father of organic chemistry
Organic chemistry lab report example
Octane lewis structure
Organic chemistry third edition david klein
Organic chemistry second edition david klein
Conjugation organic chemistry
Iupac suffix table
Carboxylic acid h3o+ reaction
Organic chemistry
Organic chemistry
Rearranged most stable carbocation is
Alkyl group example
Mass spec of chlorine
Organic chemistry
Organic chemistry cheat sheet
Organic vs inorganic compounds
This name
Meth eth prop but table
Organic synthesis via enolates
Organic chemistry
Ario organic chemistry
Organic chemistry
David klein organic chemistry
What is organic chemistry like
The art of writing reasonable organic reaction mechanisms
Eth meth prop but pent
Canola oil
Alkane organic chemistry
Oxidation of carbohydrates
Iupac
Organic chemistry
Organic chemistry chapter 9
Organic chemistry
Extraction of caffeine from vivarin tablets lab report