Electrochemistry Galvanic cells Cell Potentials and Standard cell
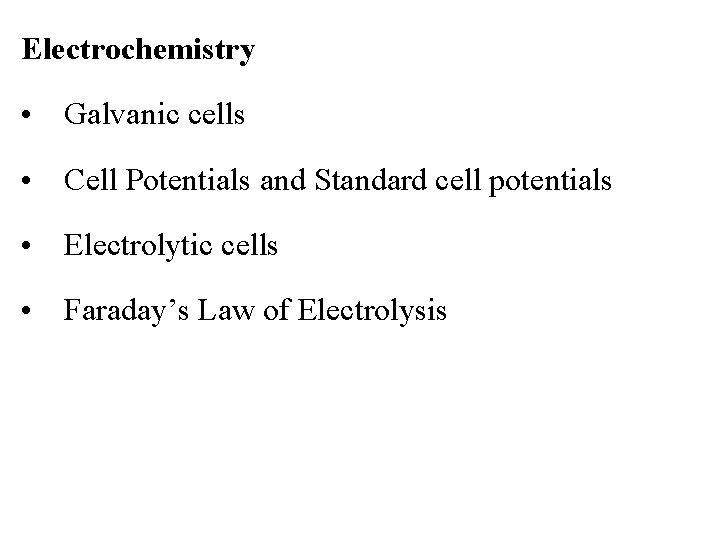
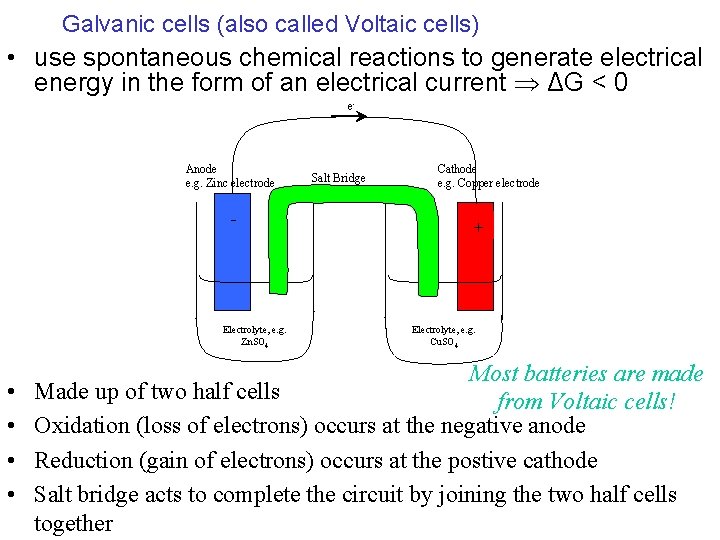
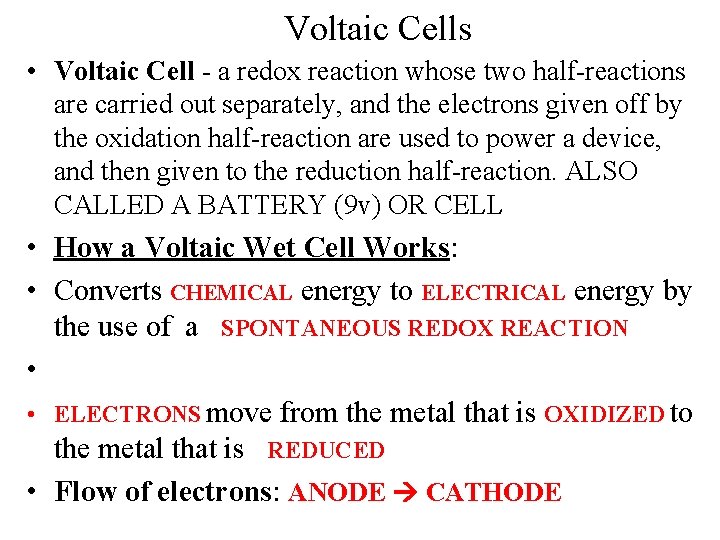
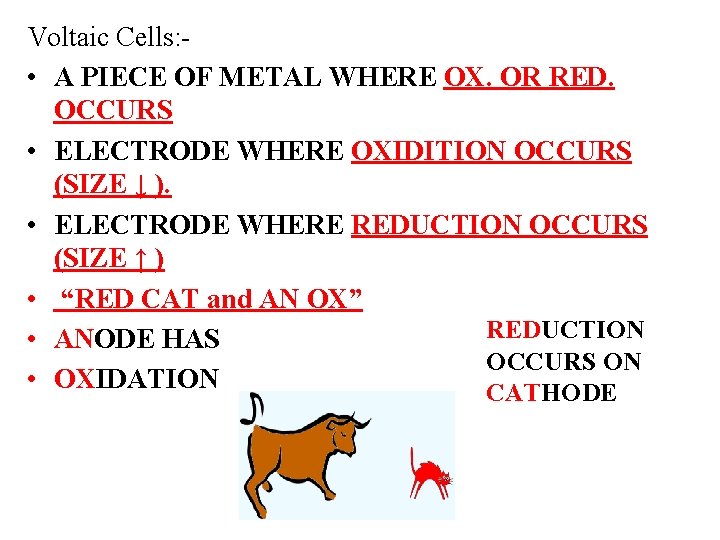
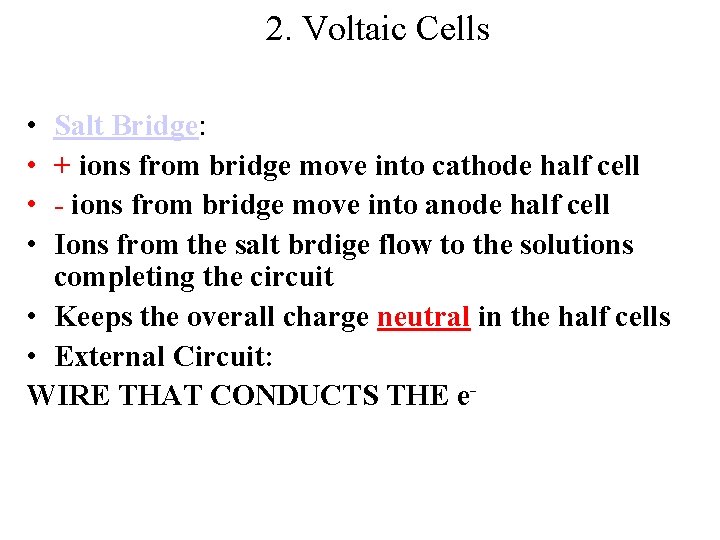
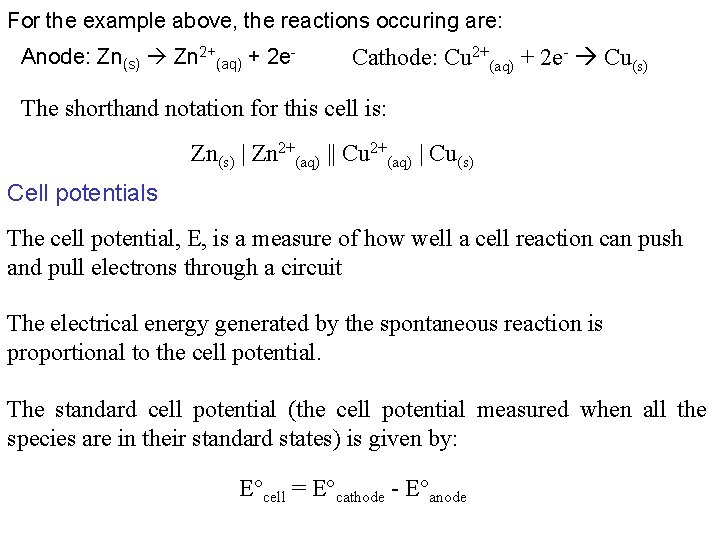
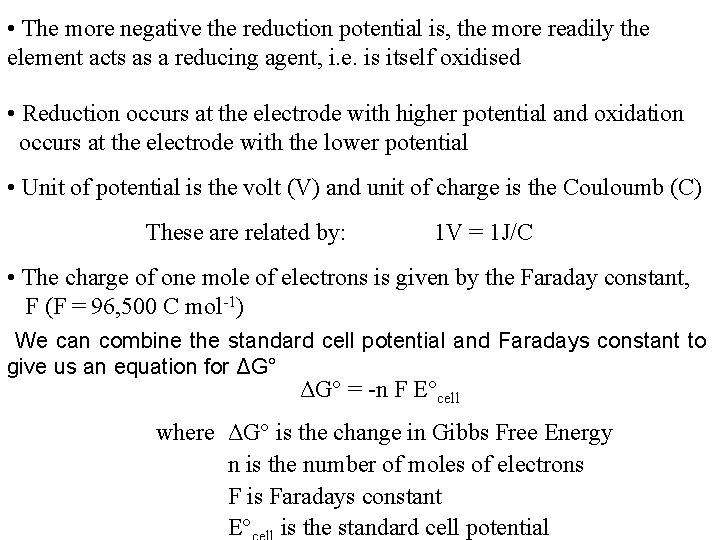
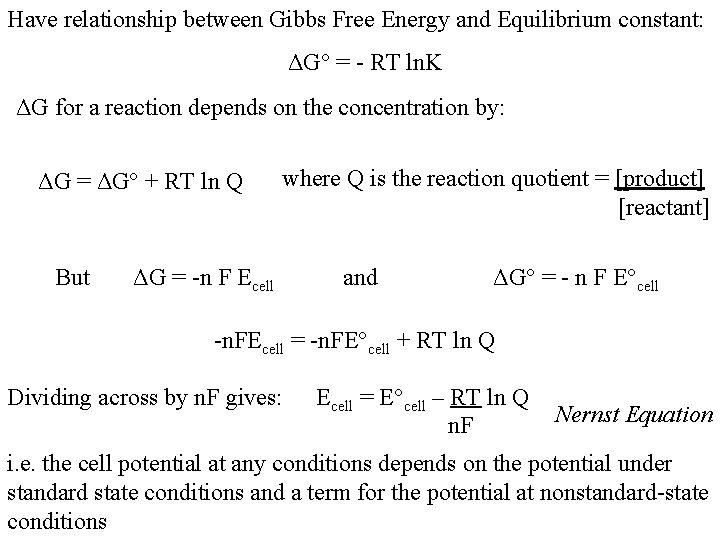
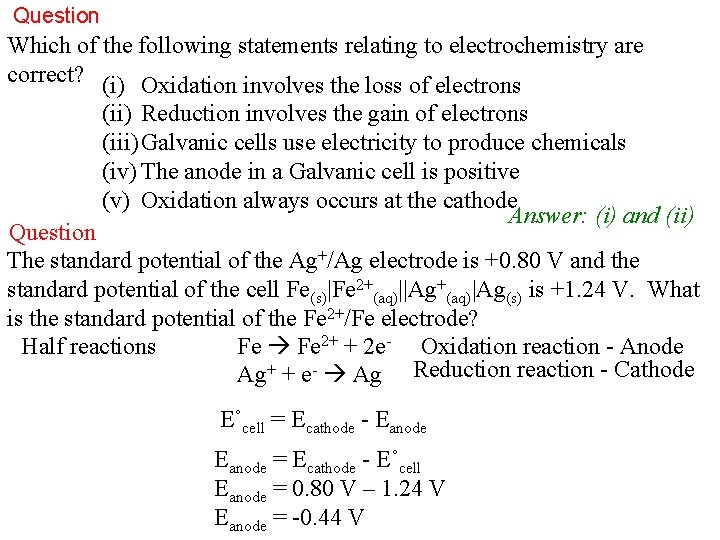
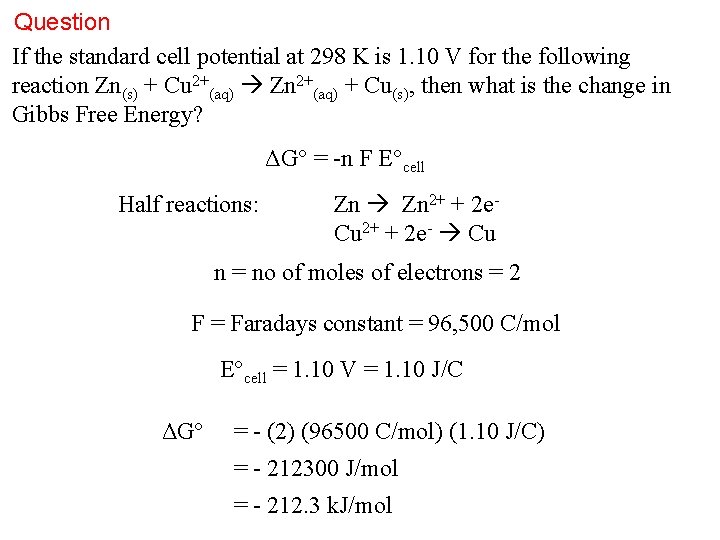
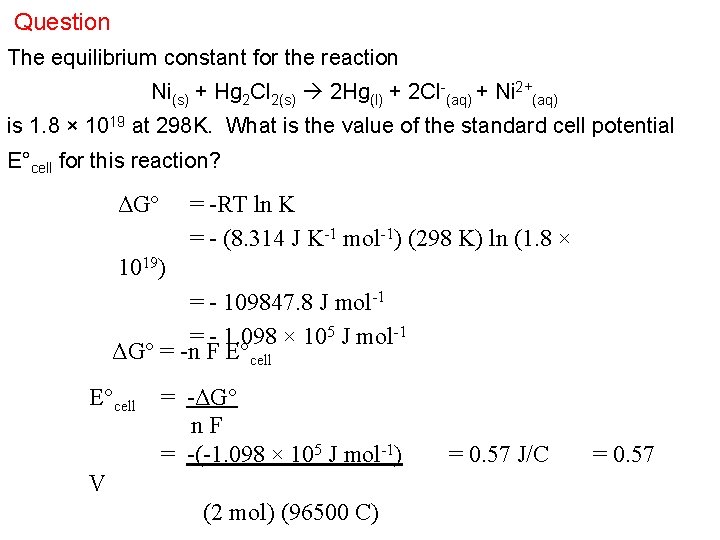
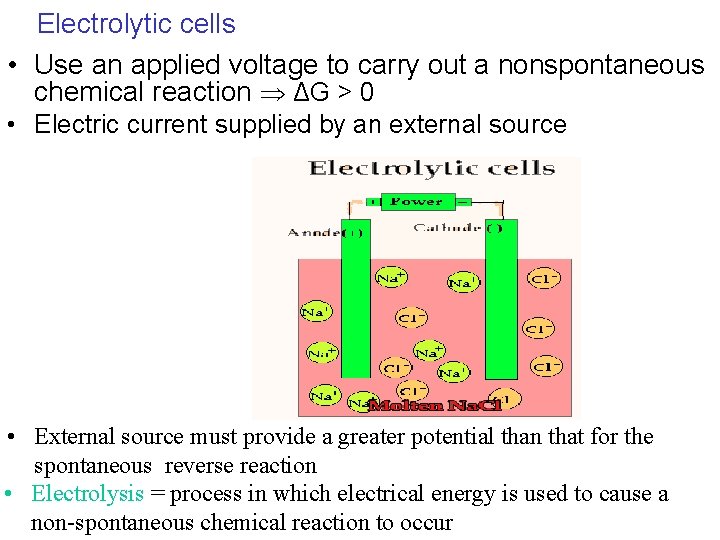
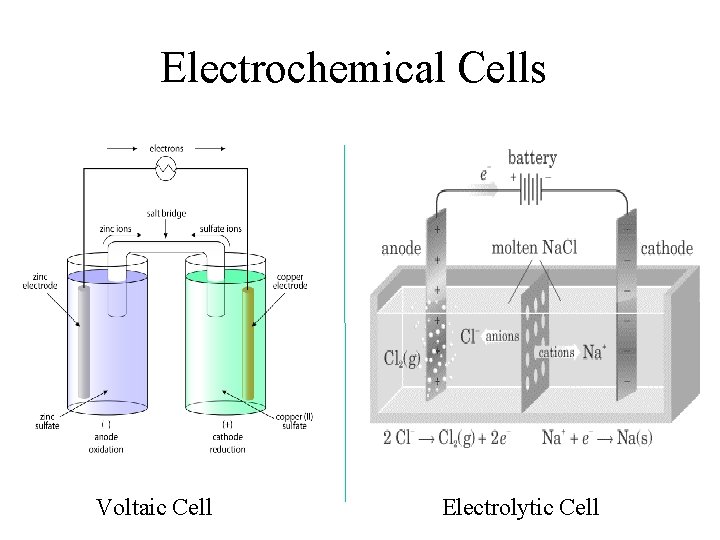
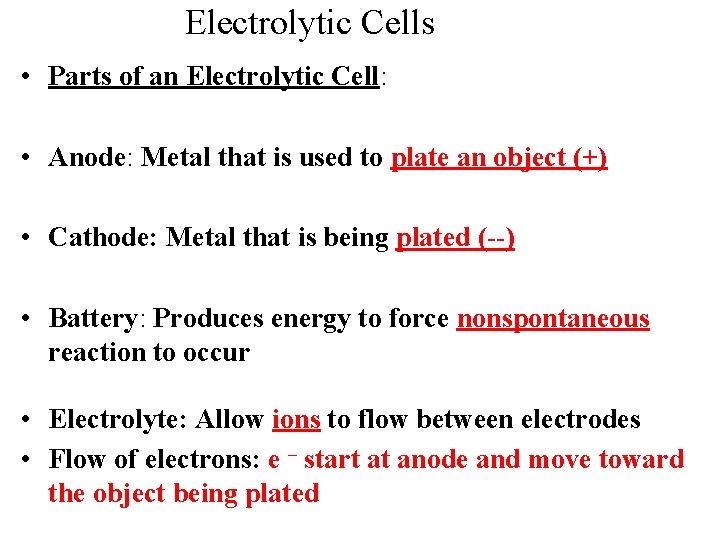
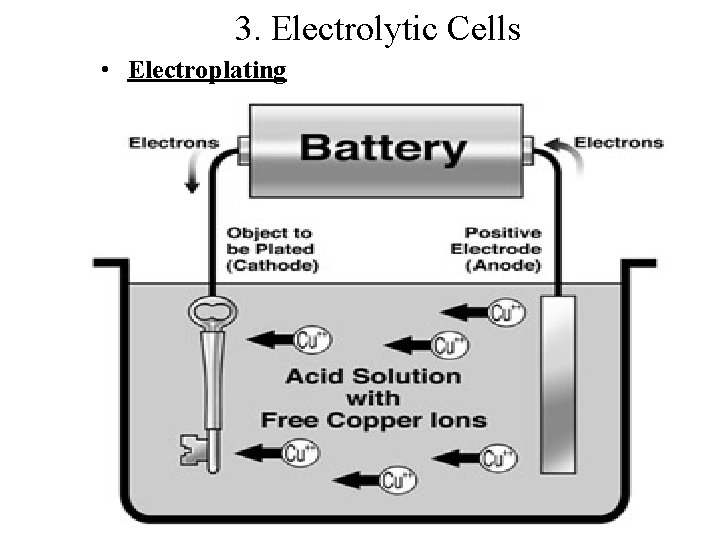
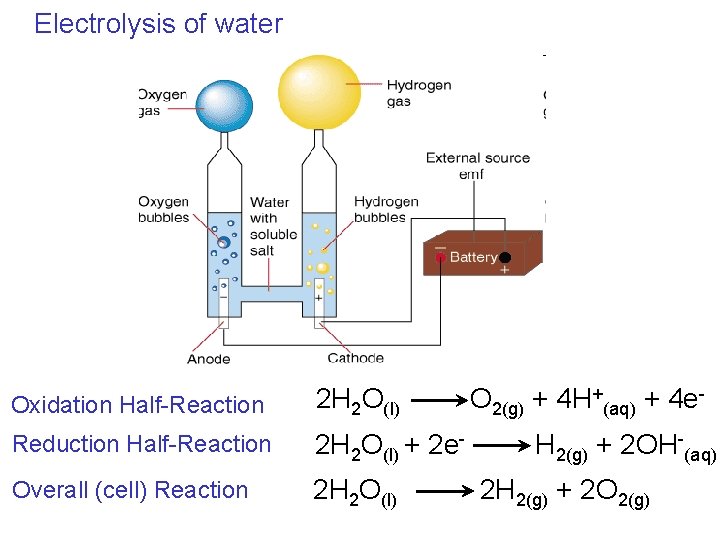
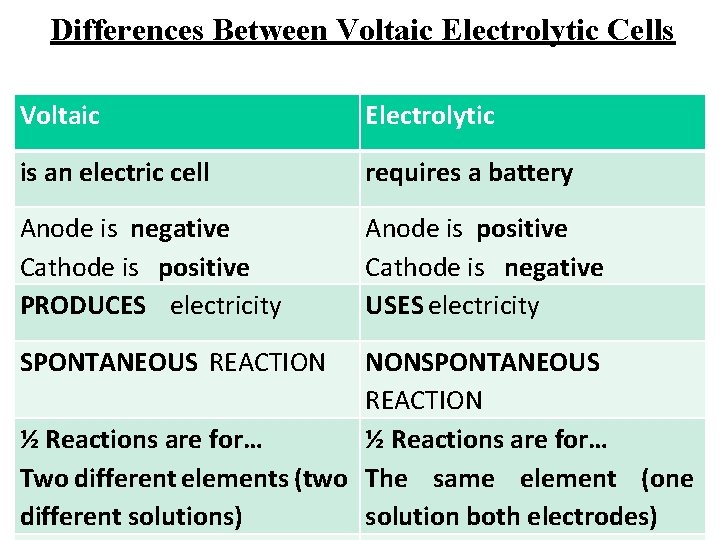
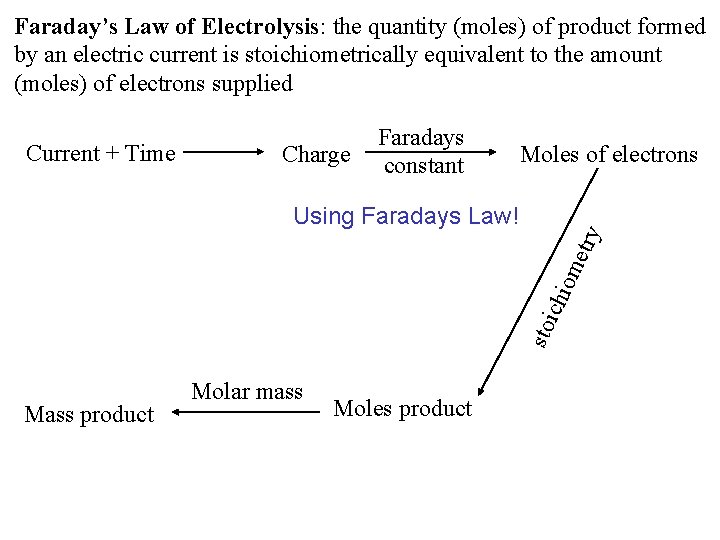
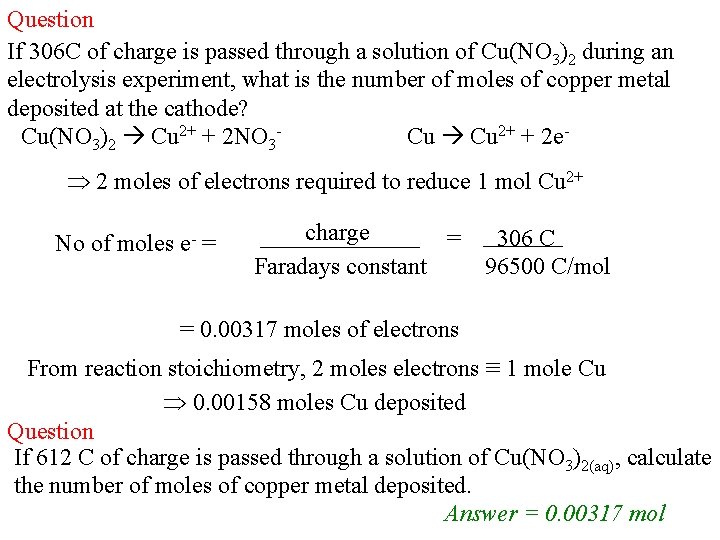
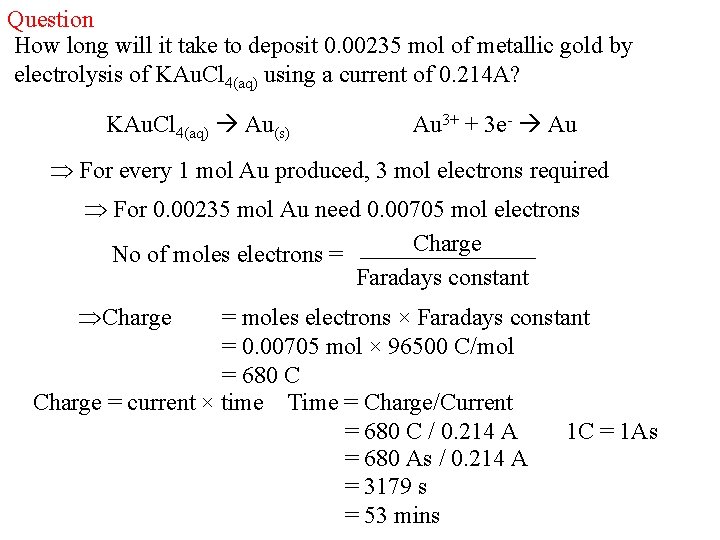
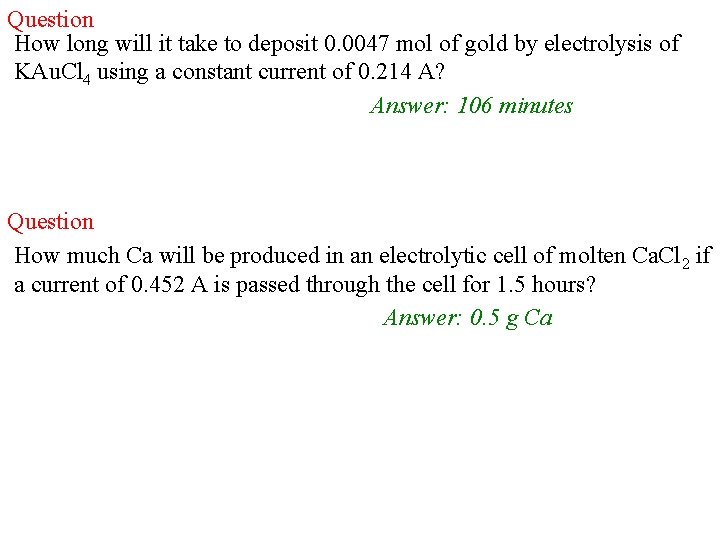
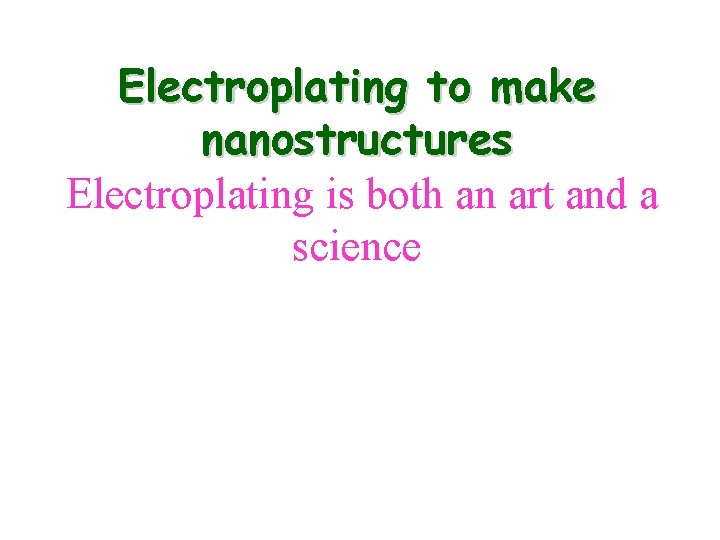
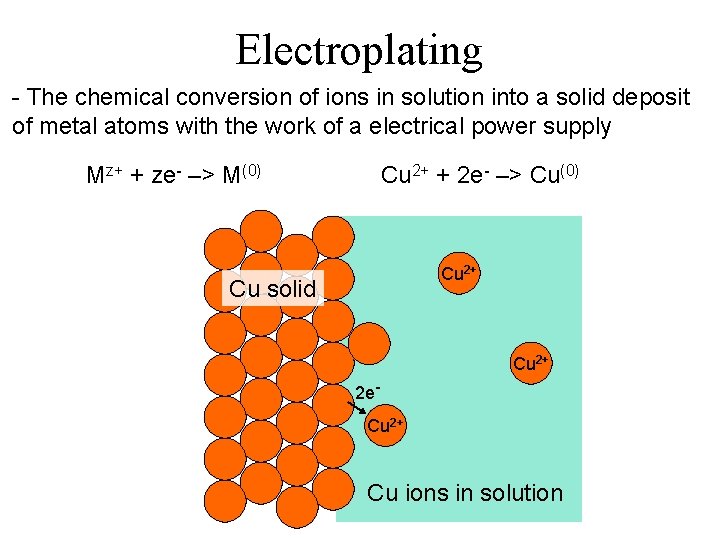
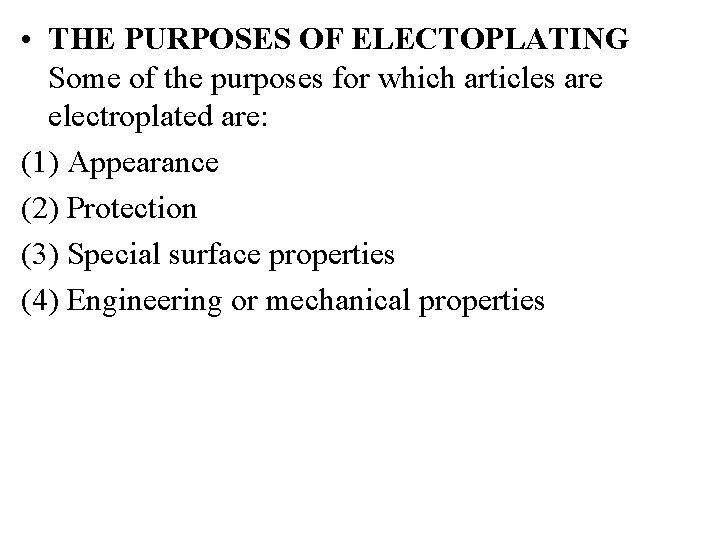
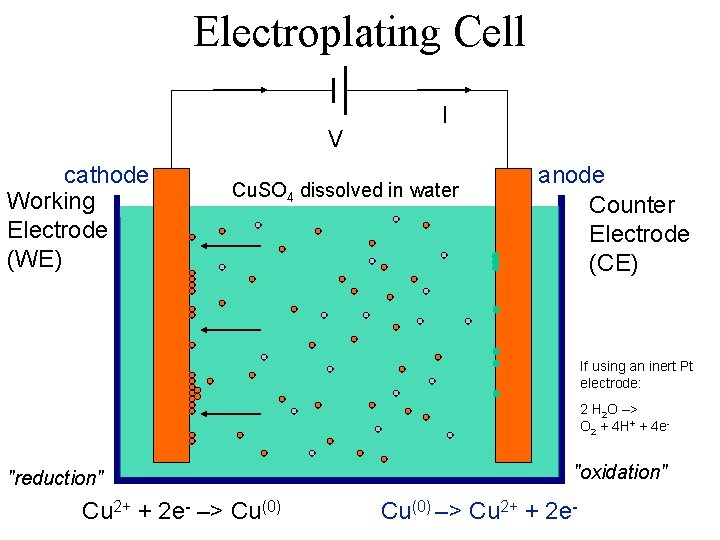
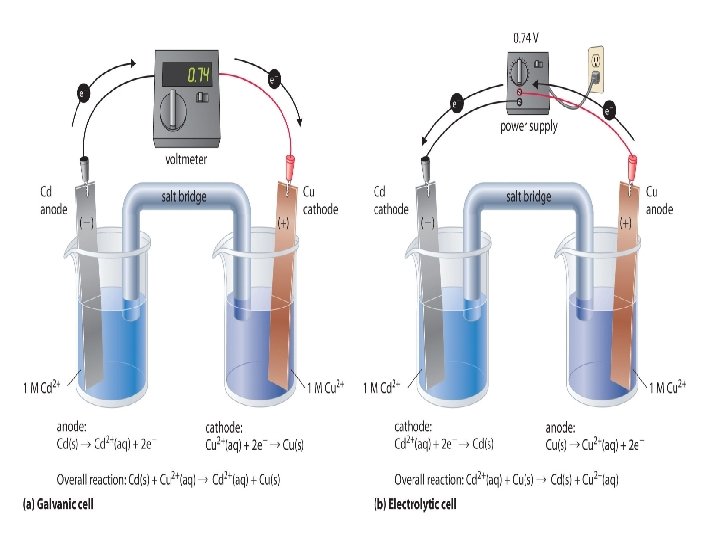
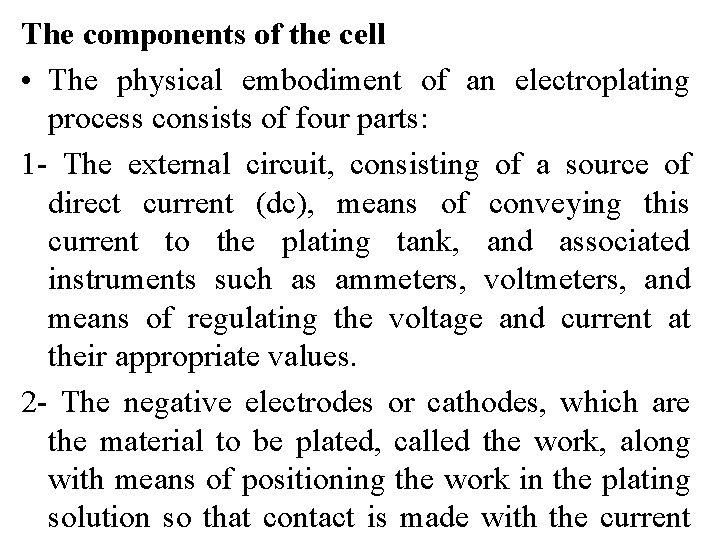
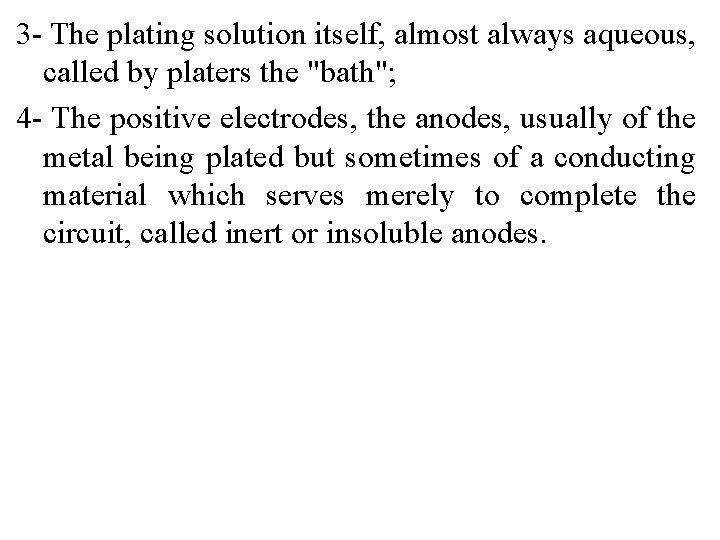
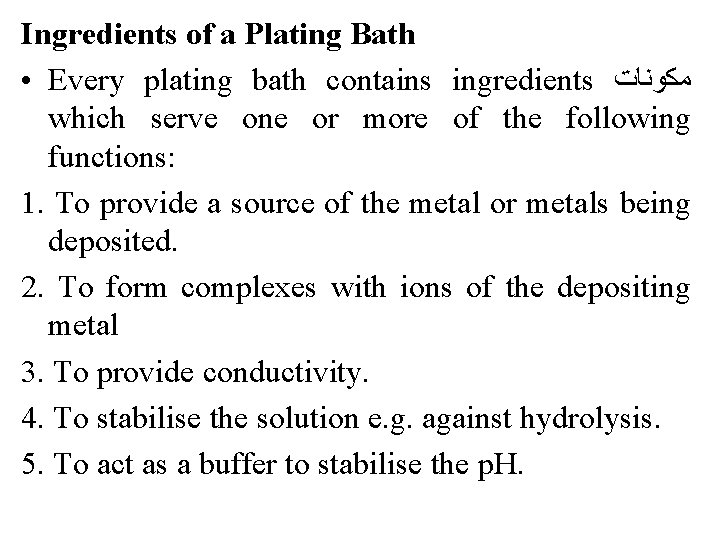
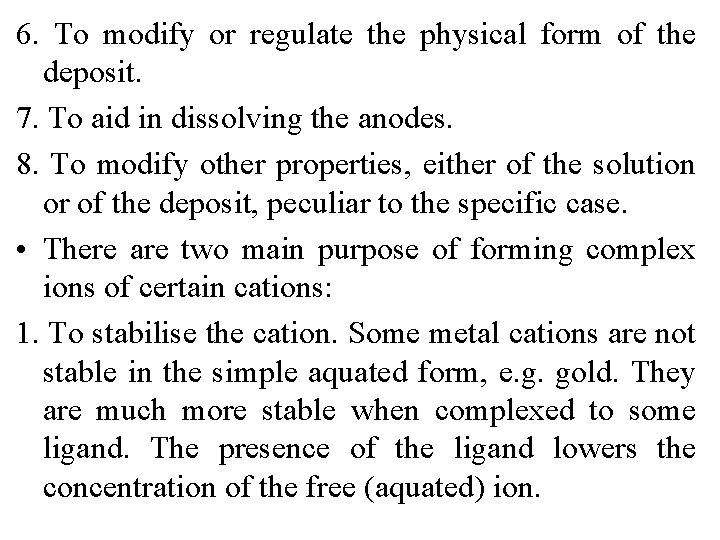
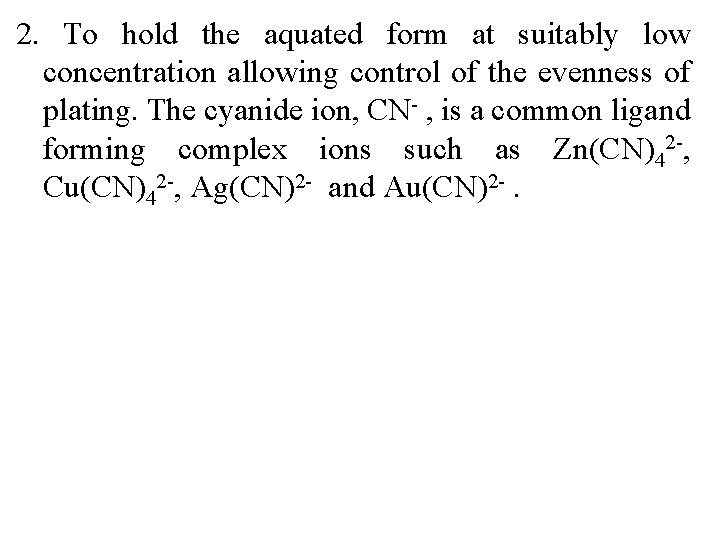
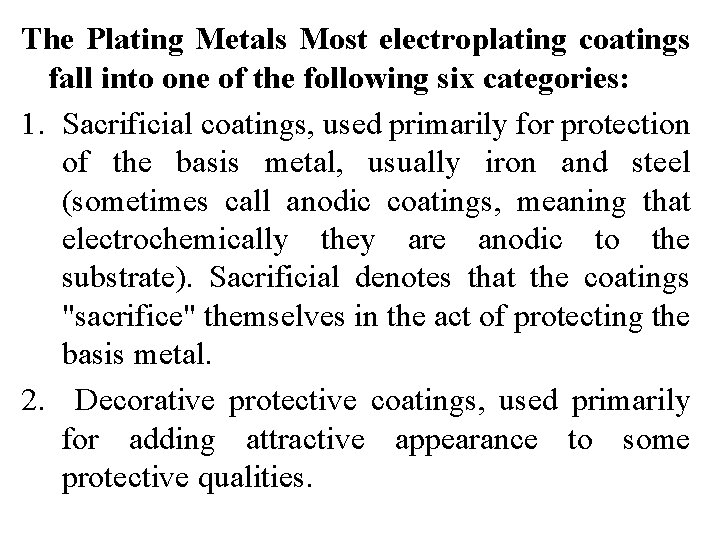
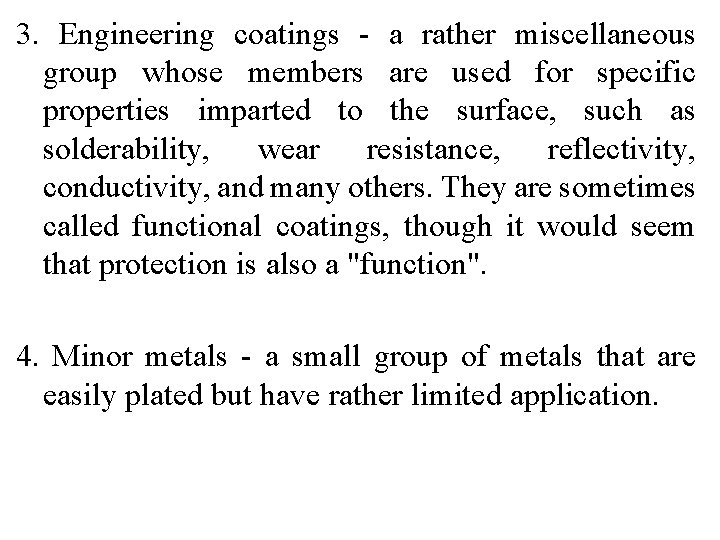
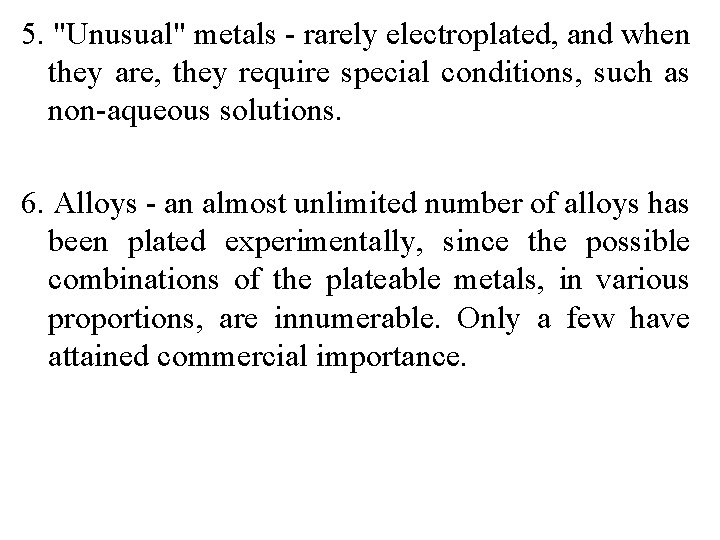
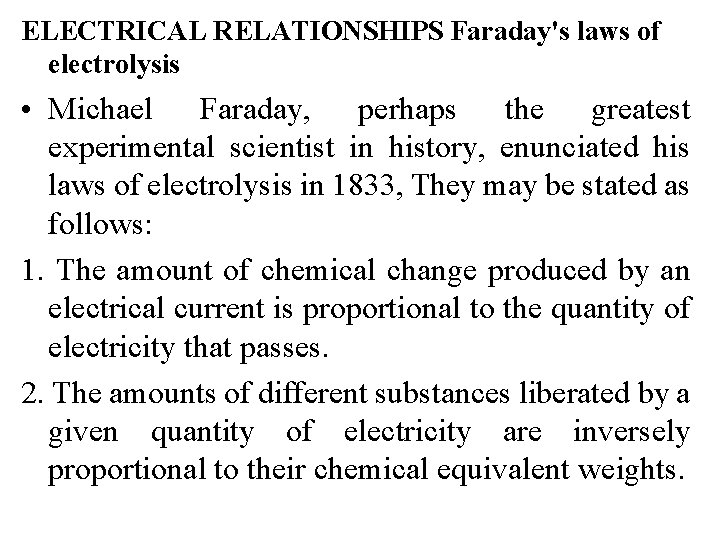
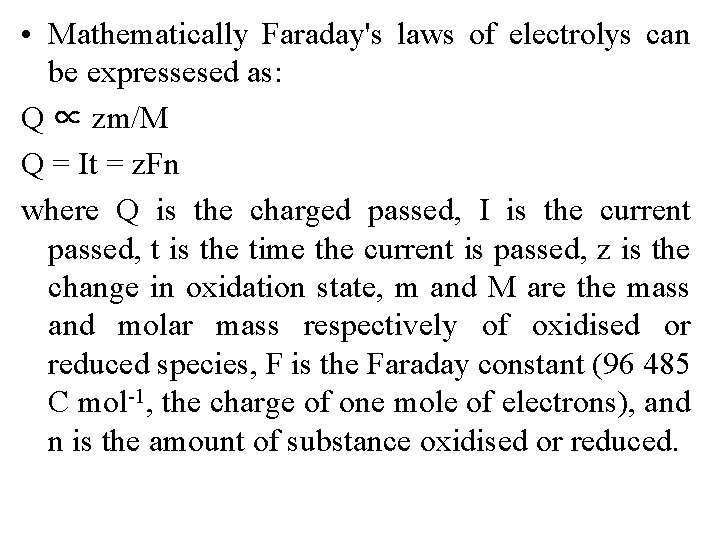
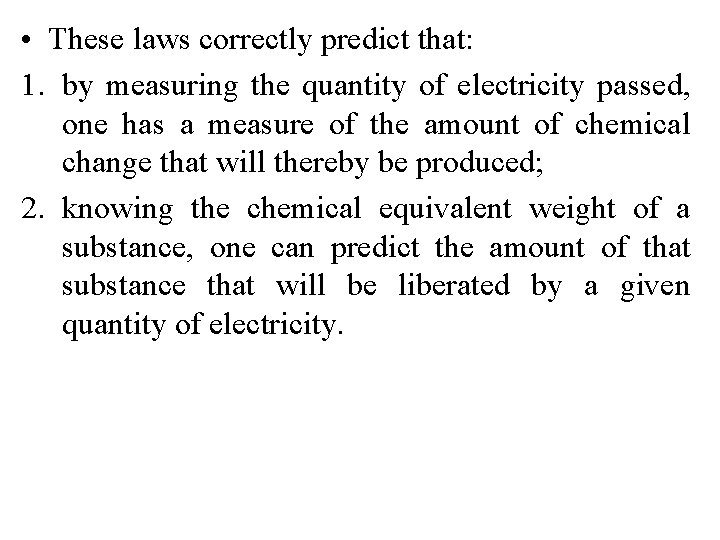
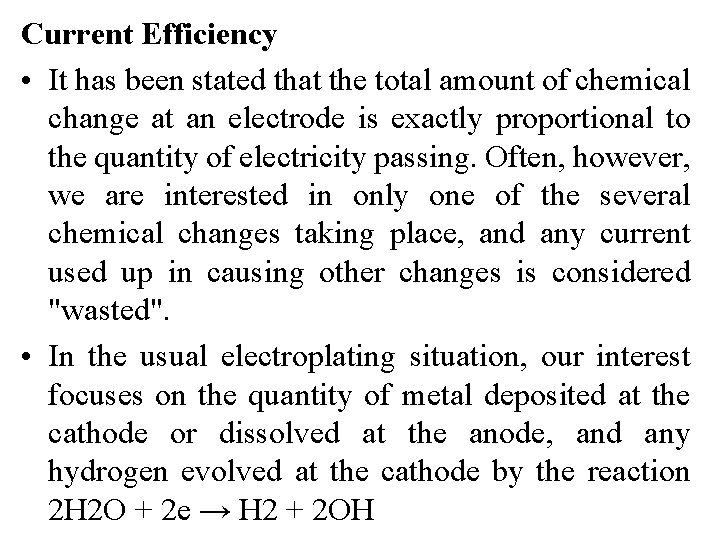
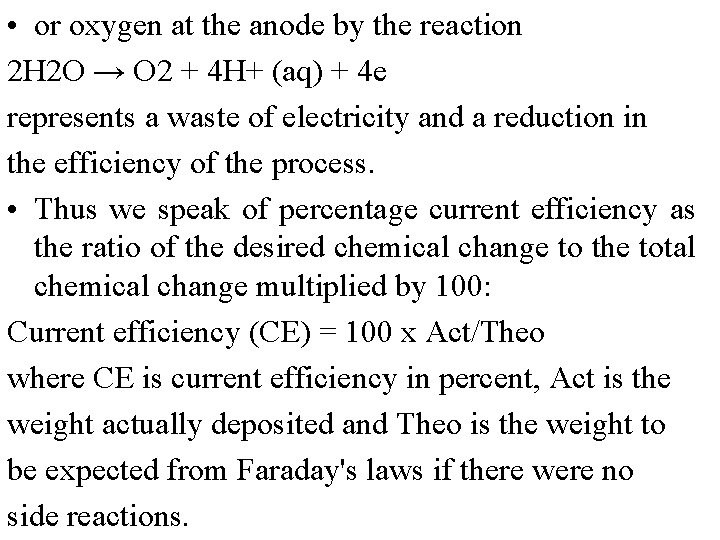
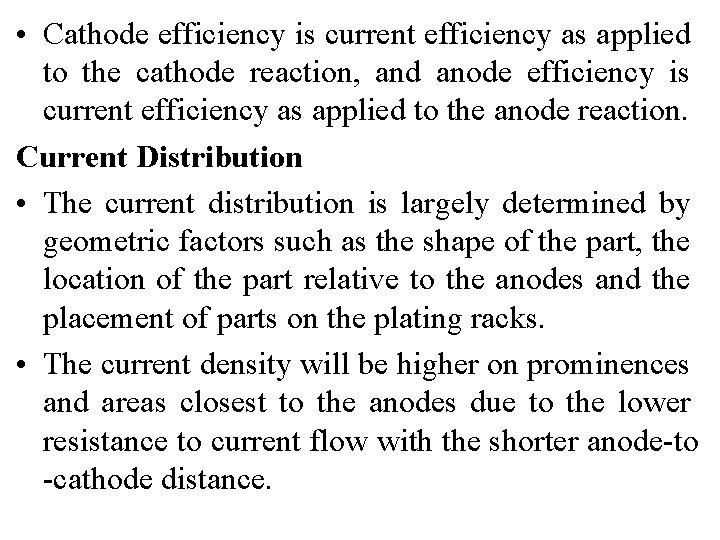
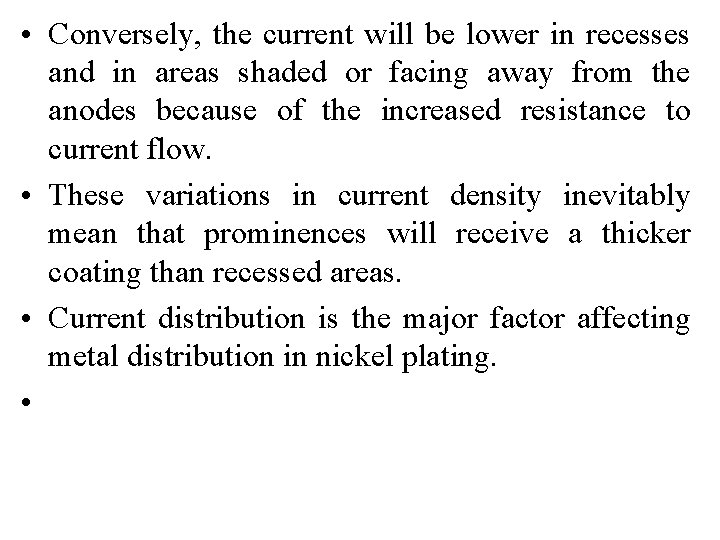
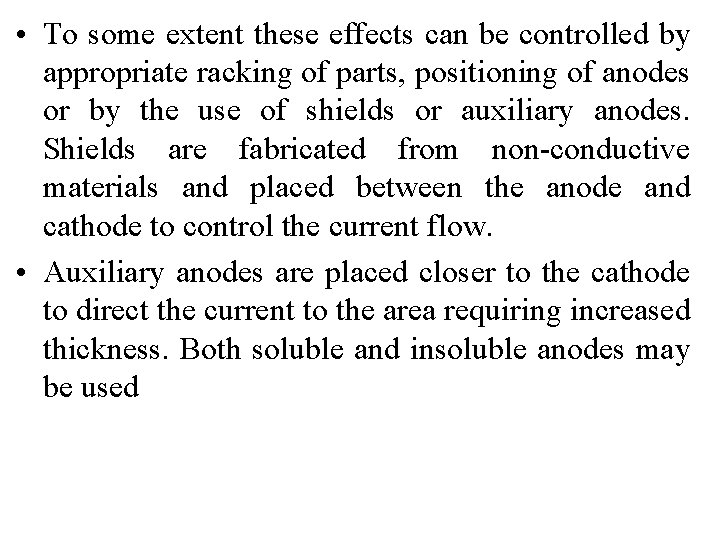
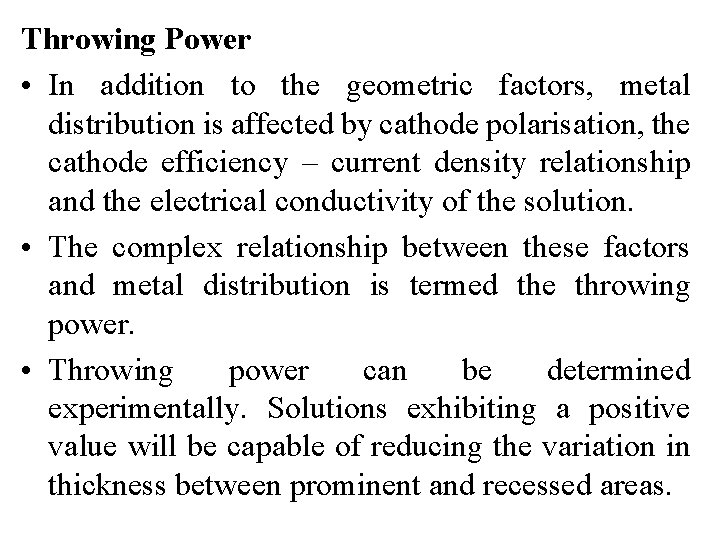
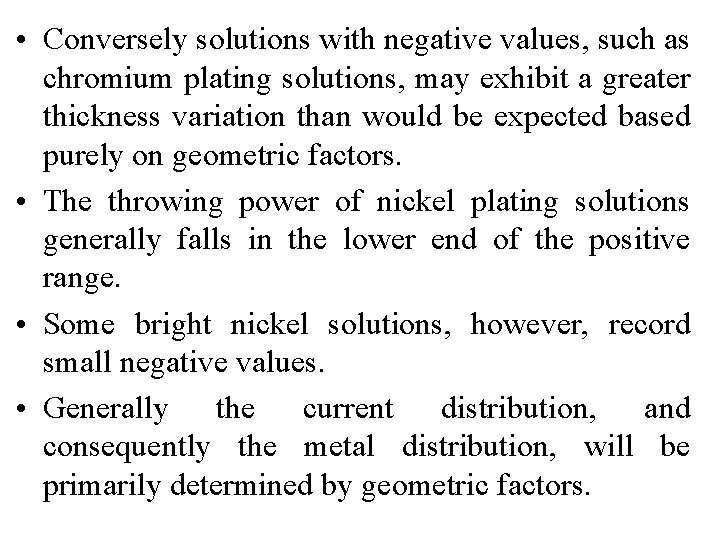
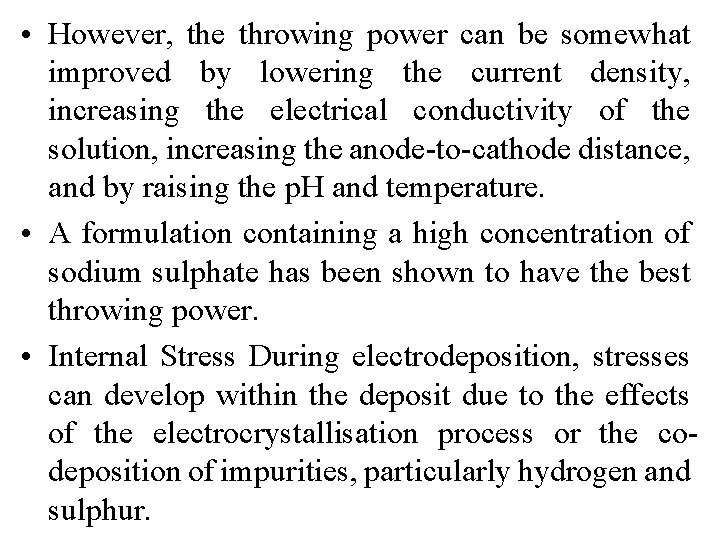
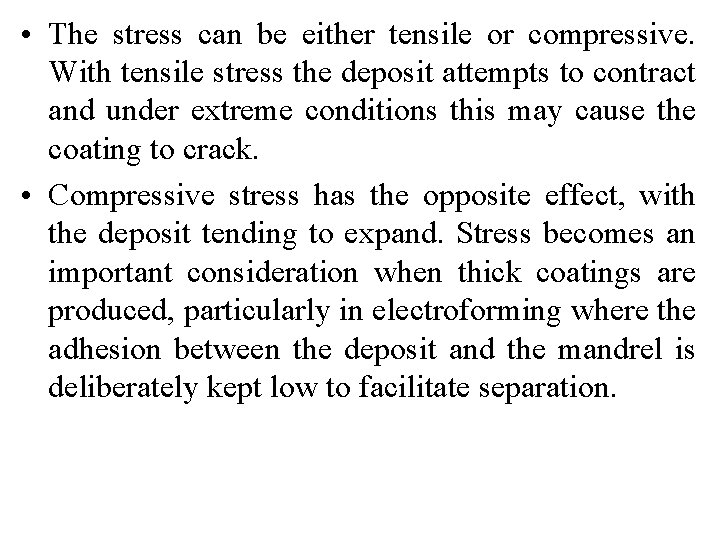
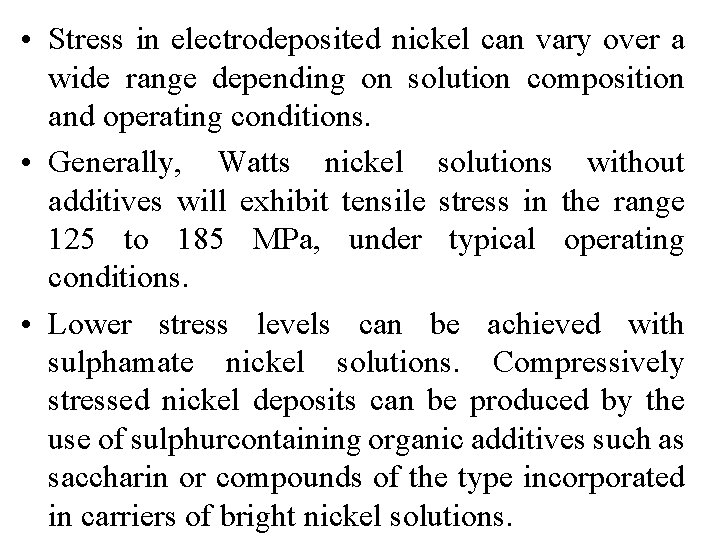
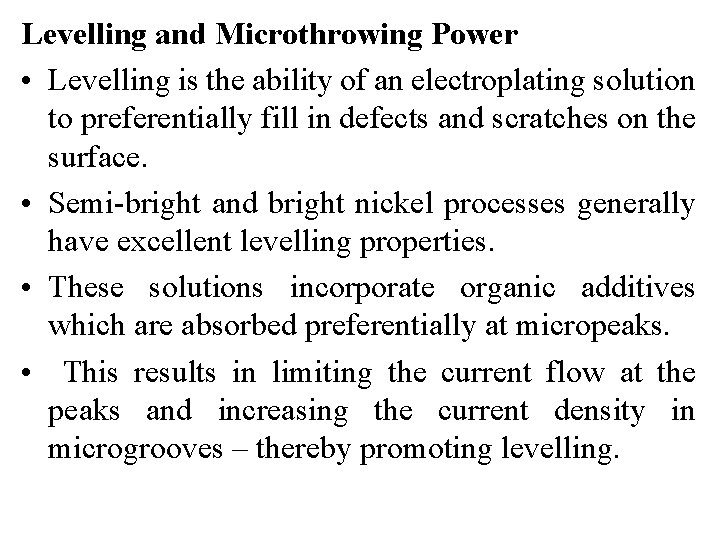
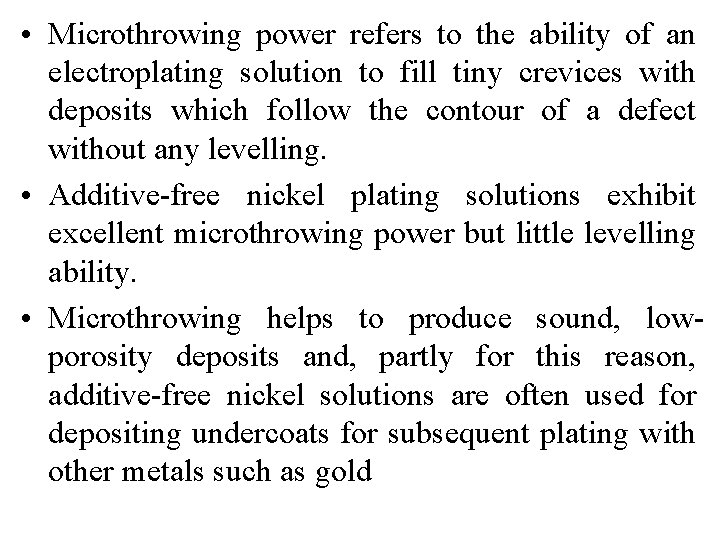
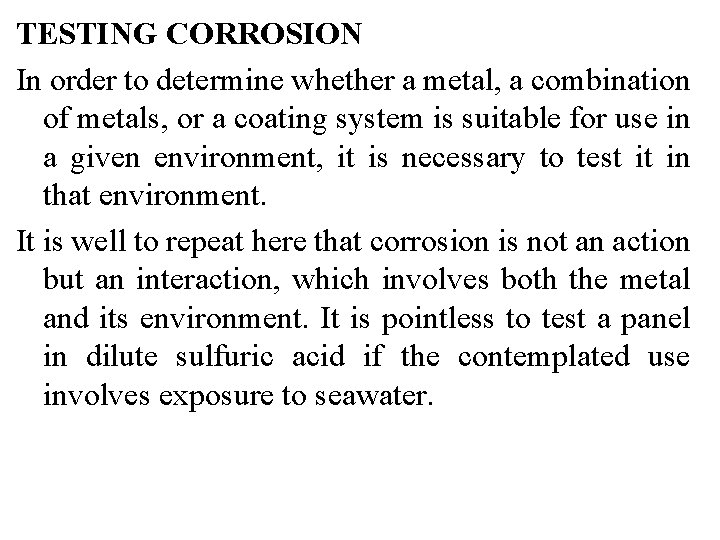
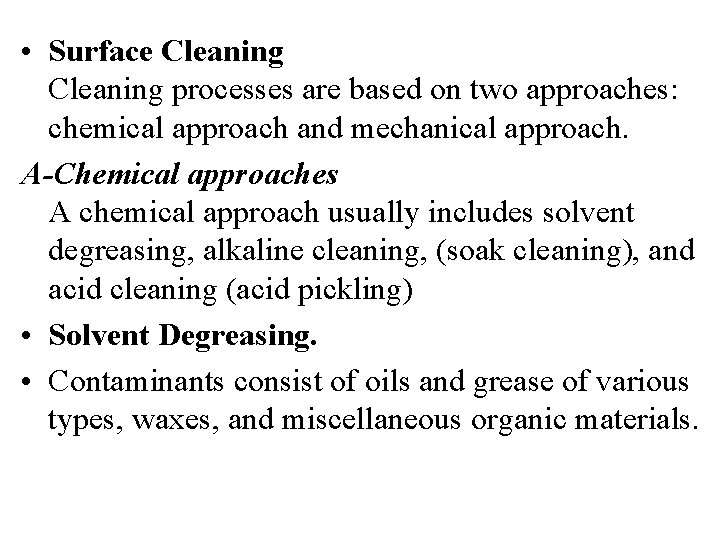
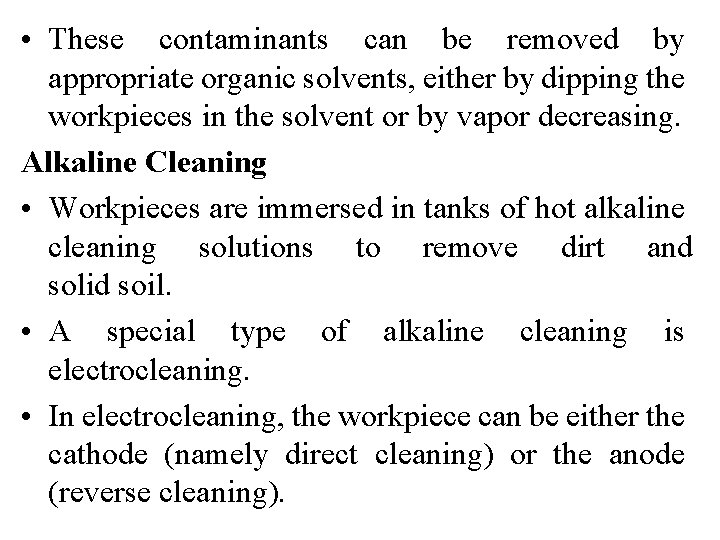
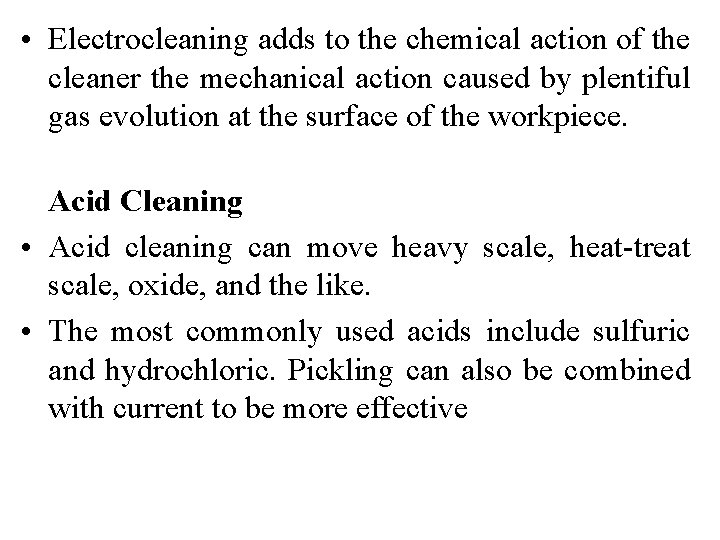
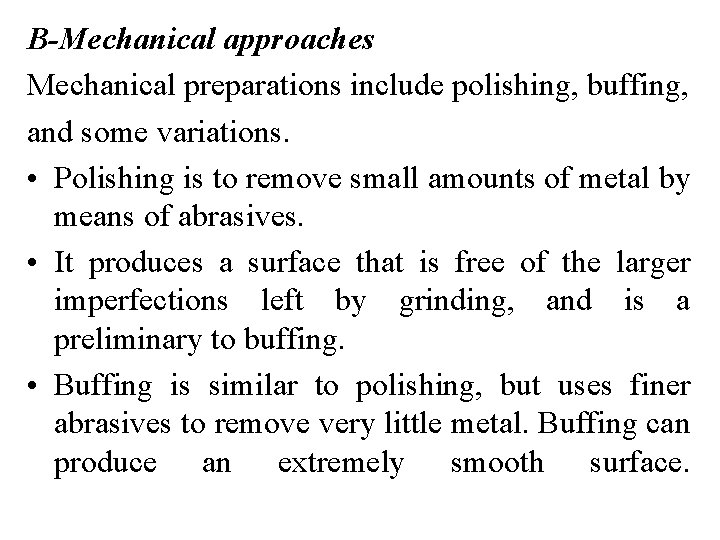
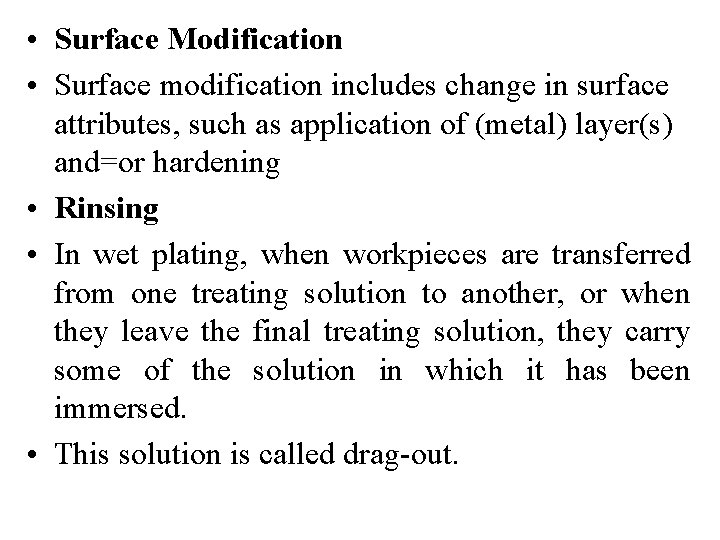
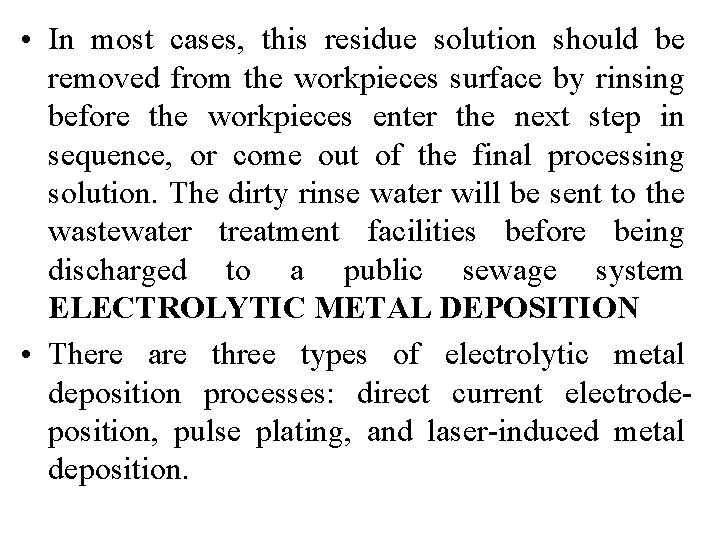
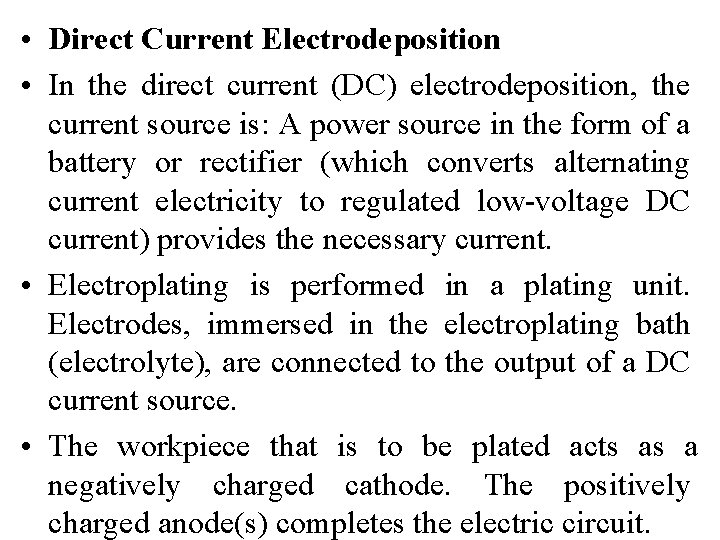
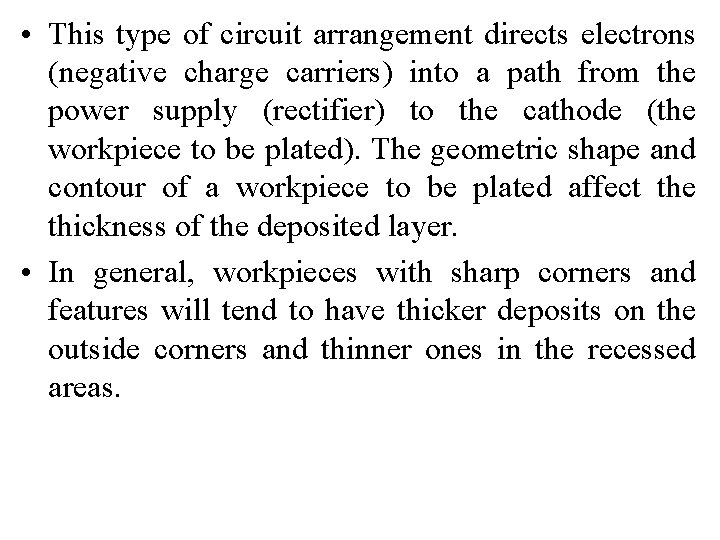
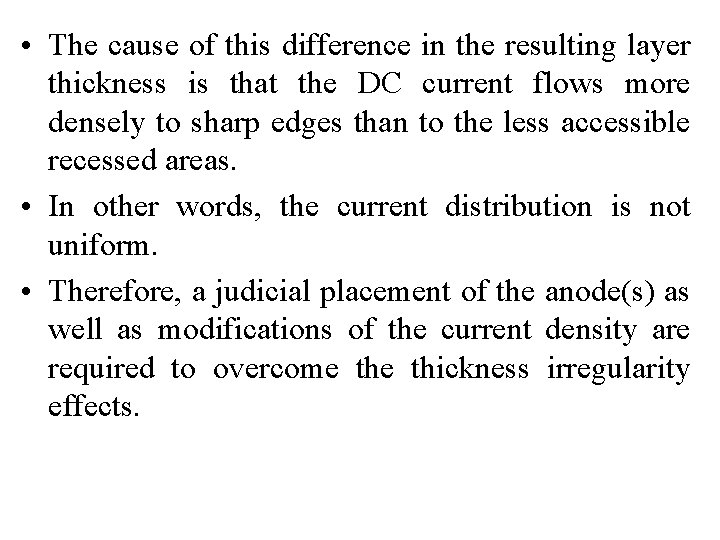
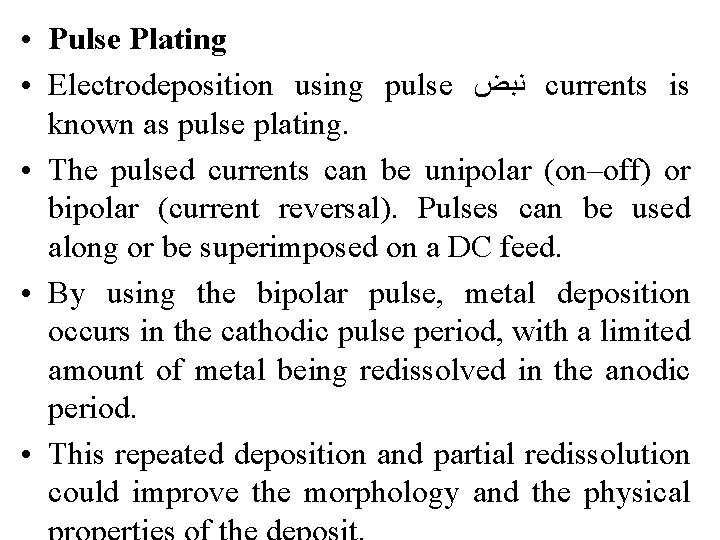
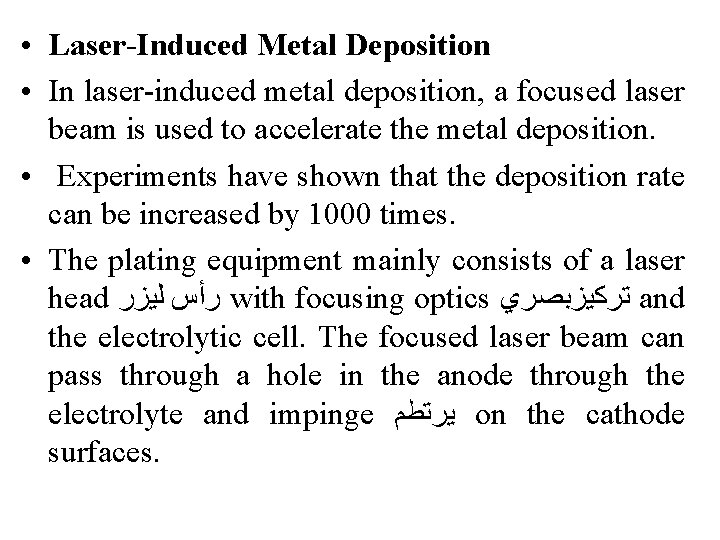
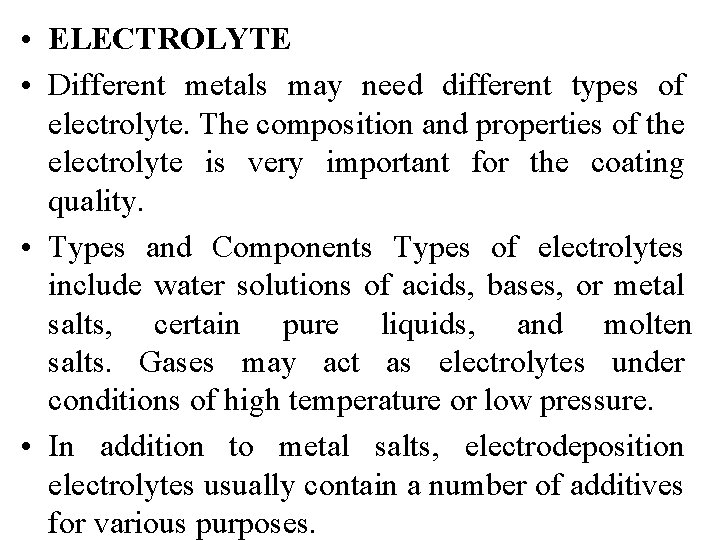
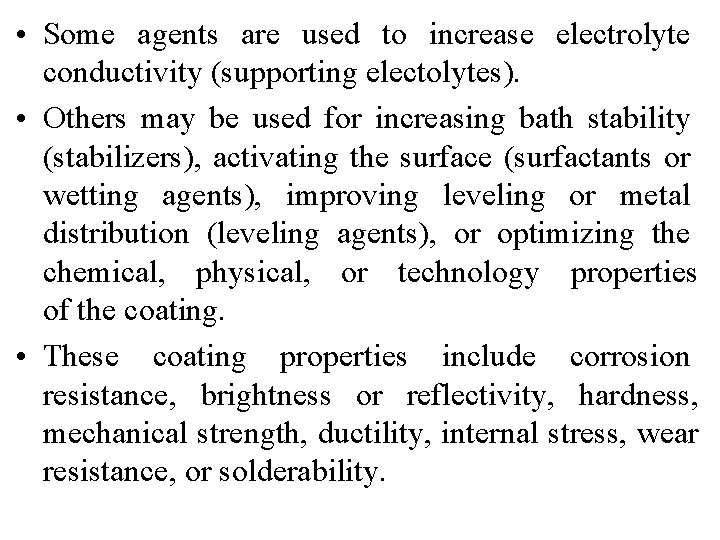
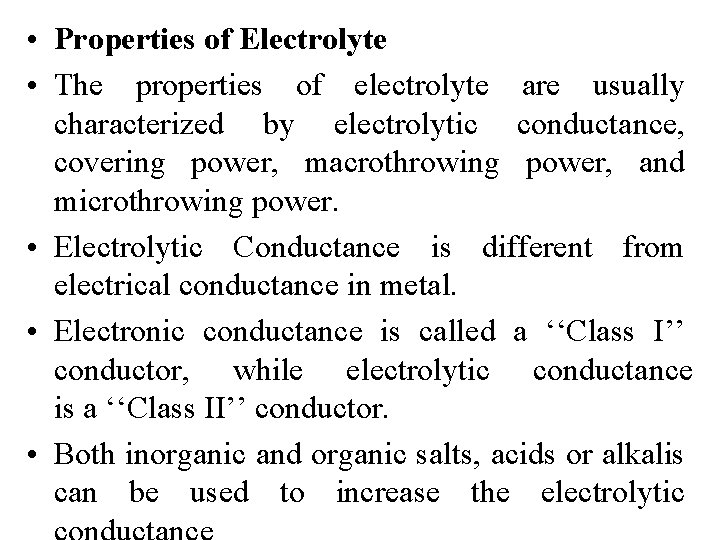
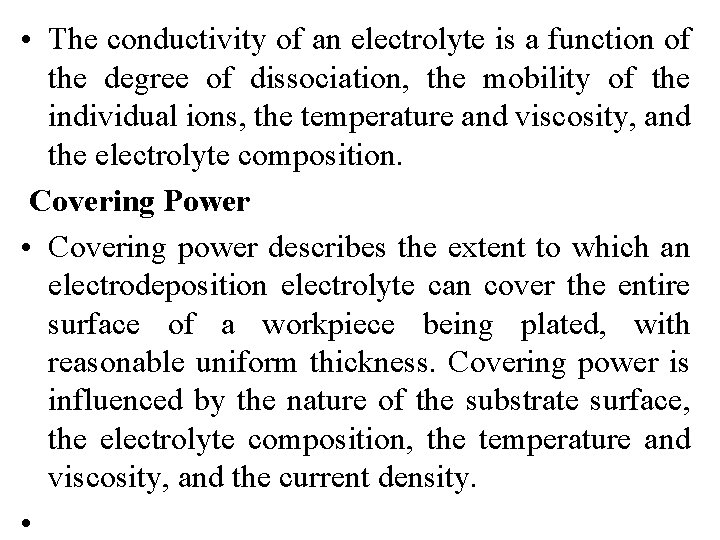
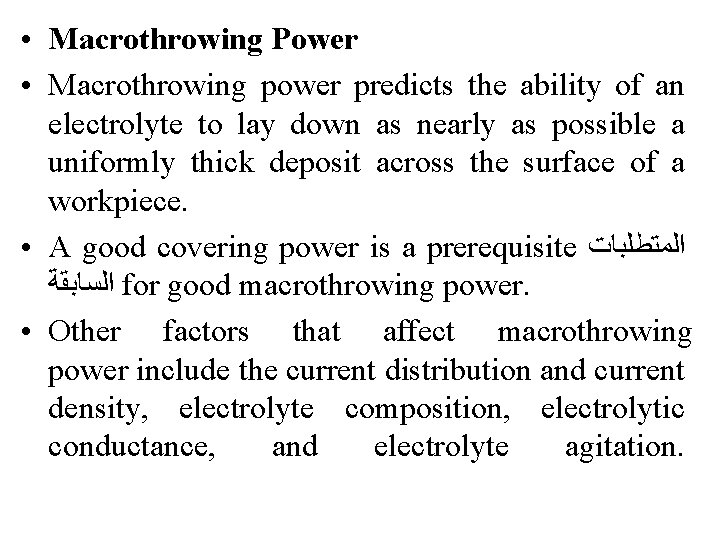
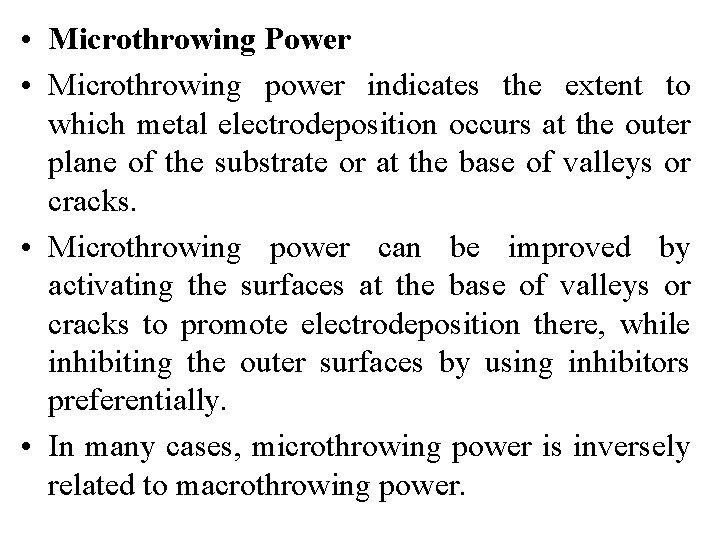
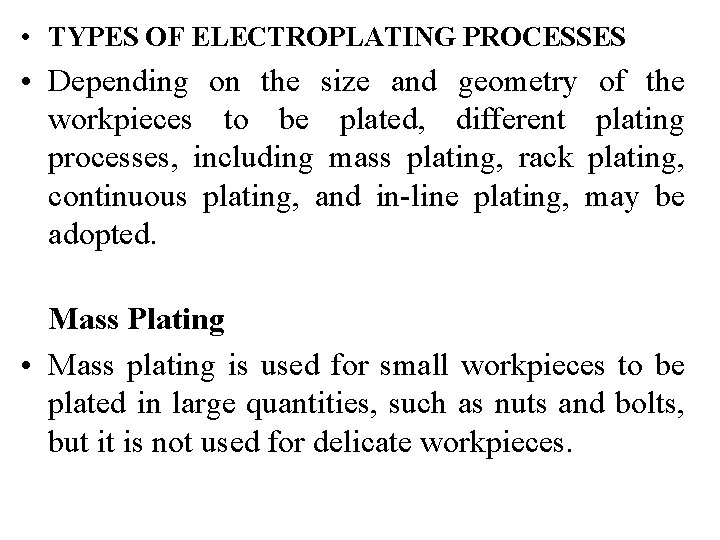
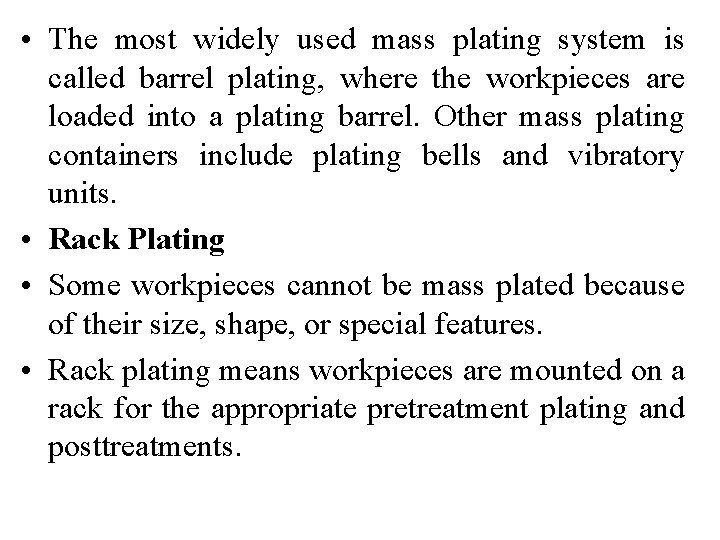
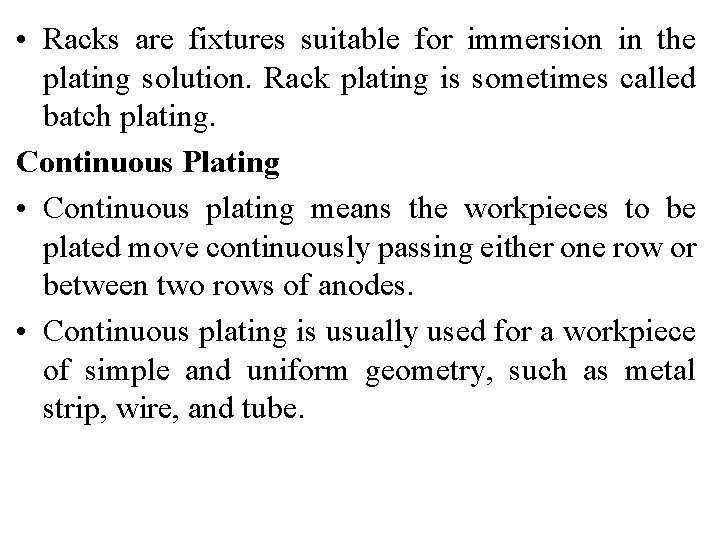
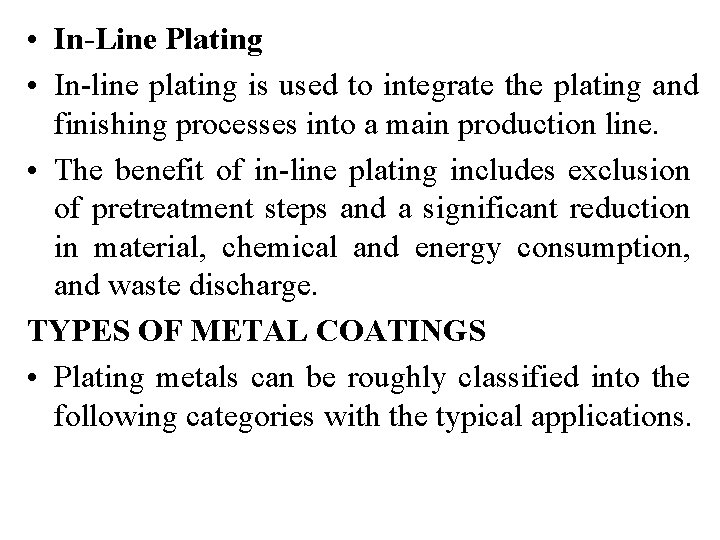
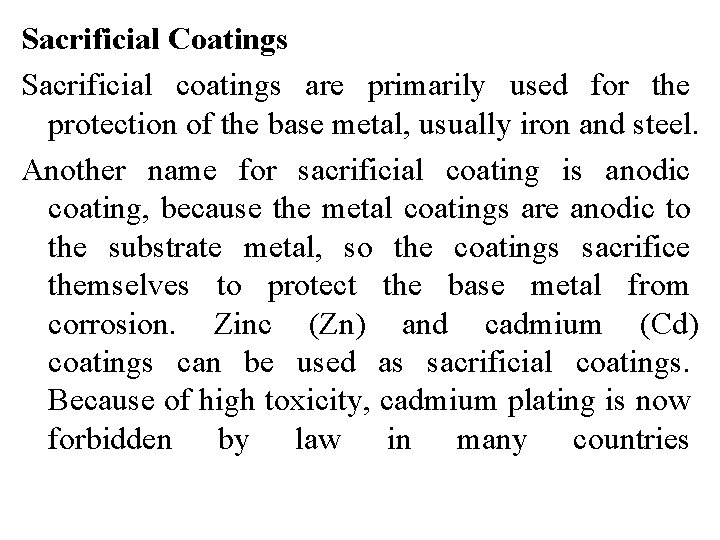
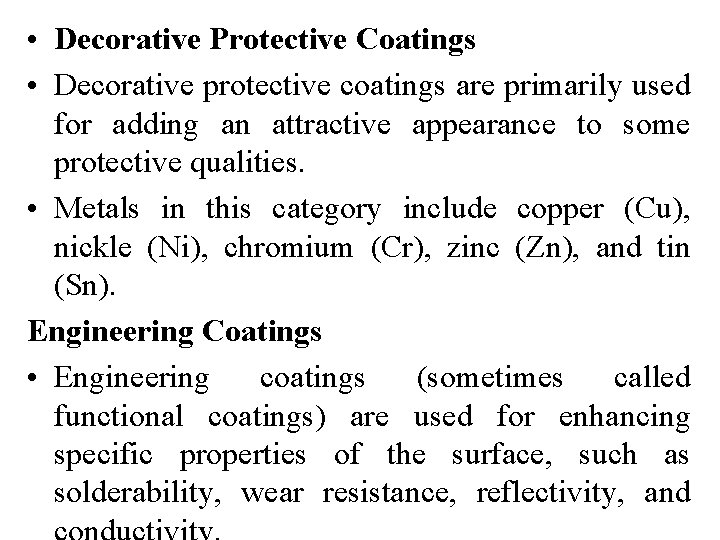
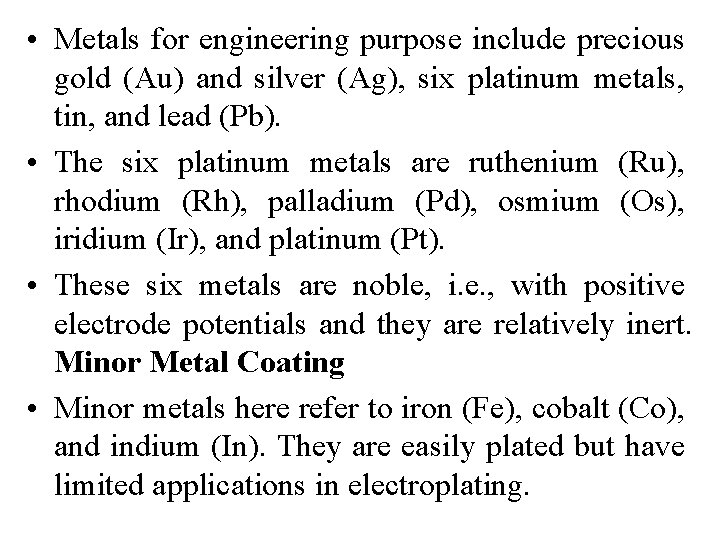
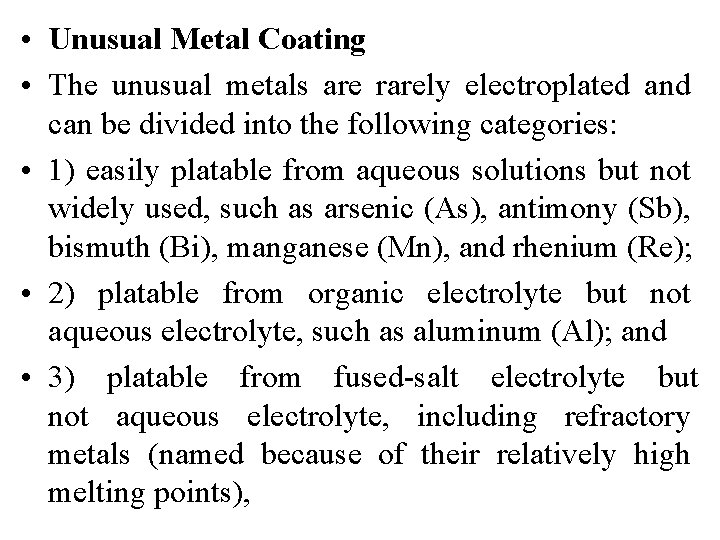
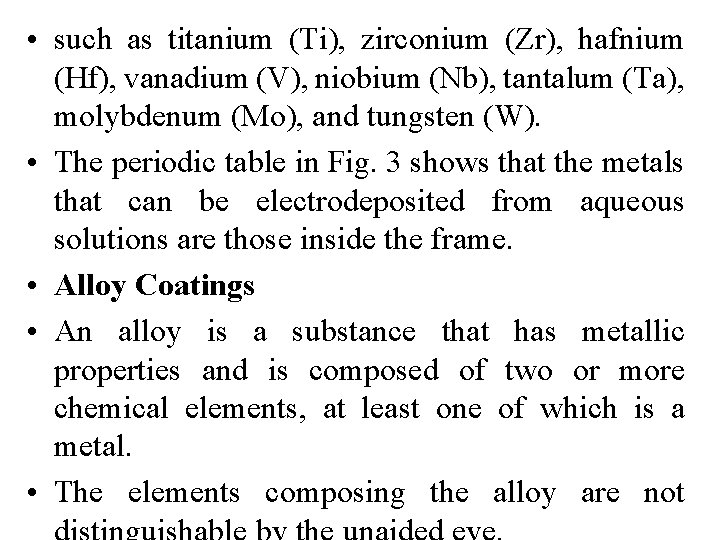
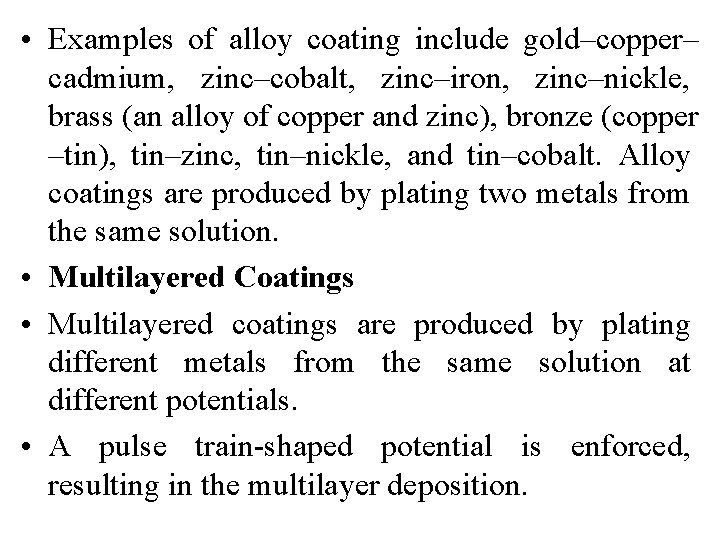
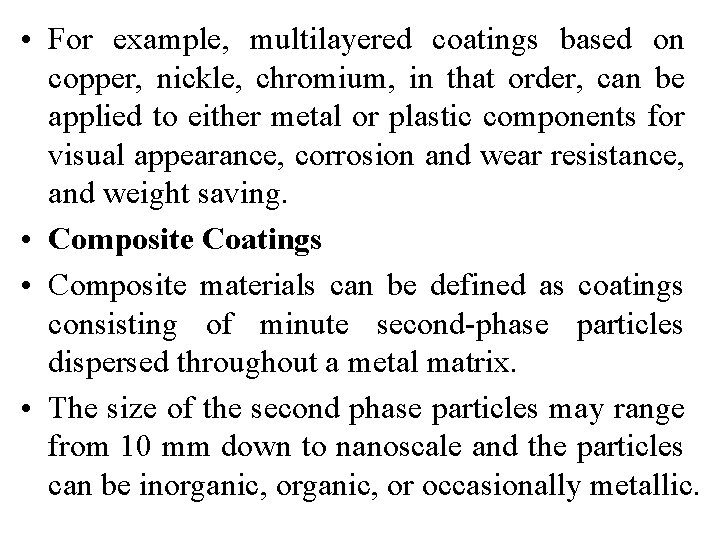
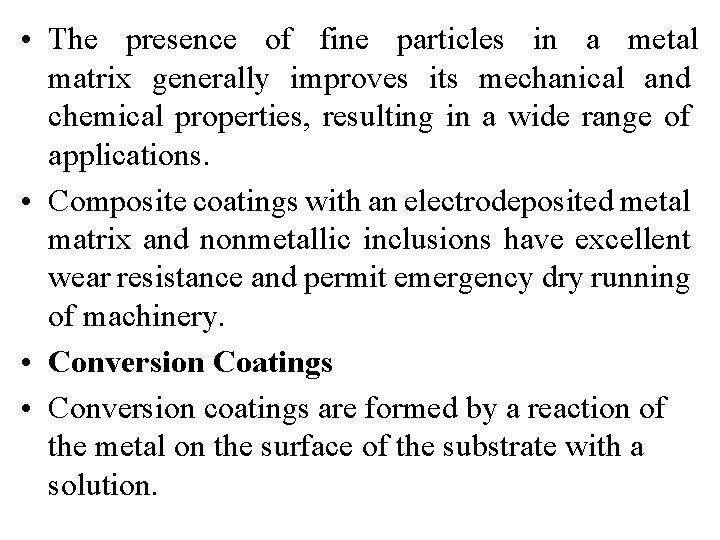
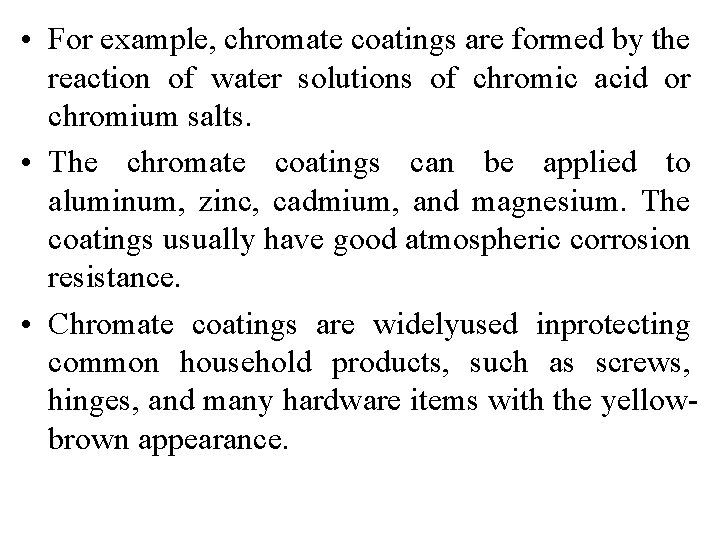
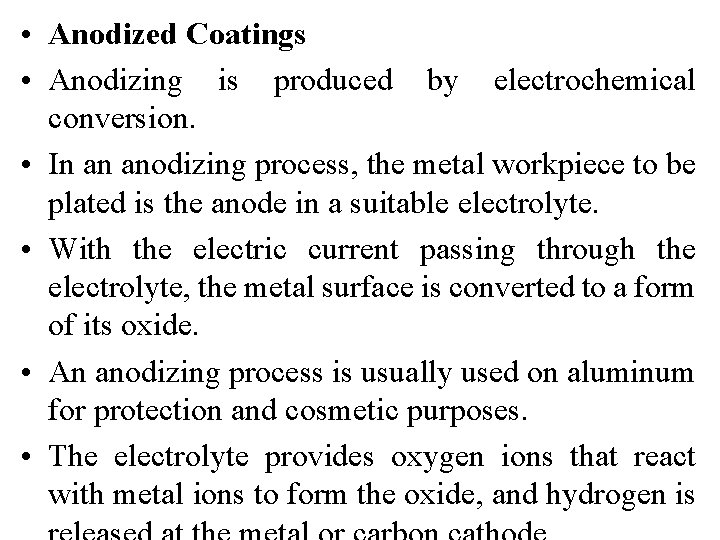
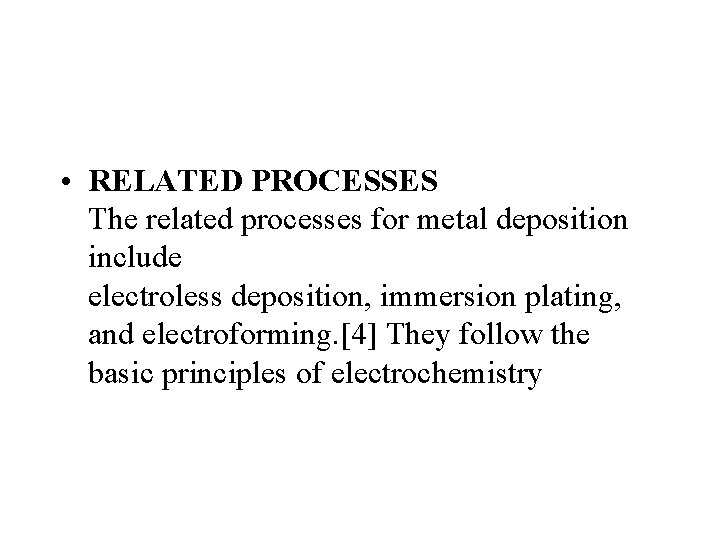
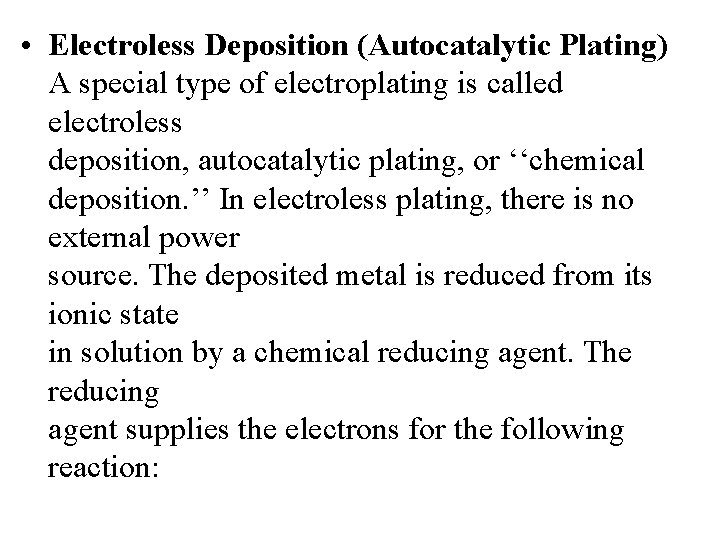
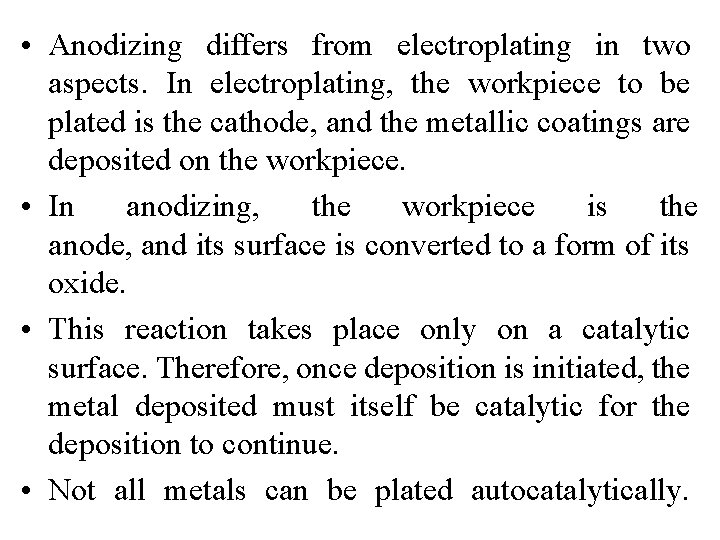
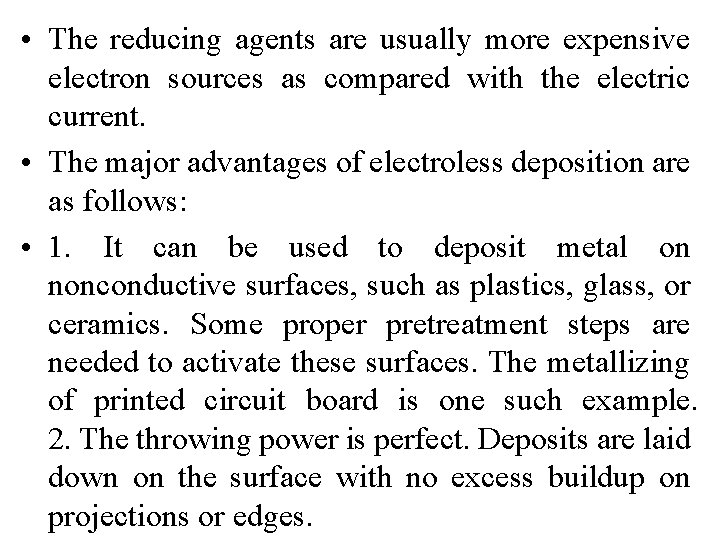
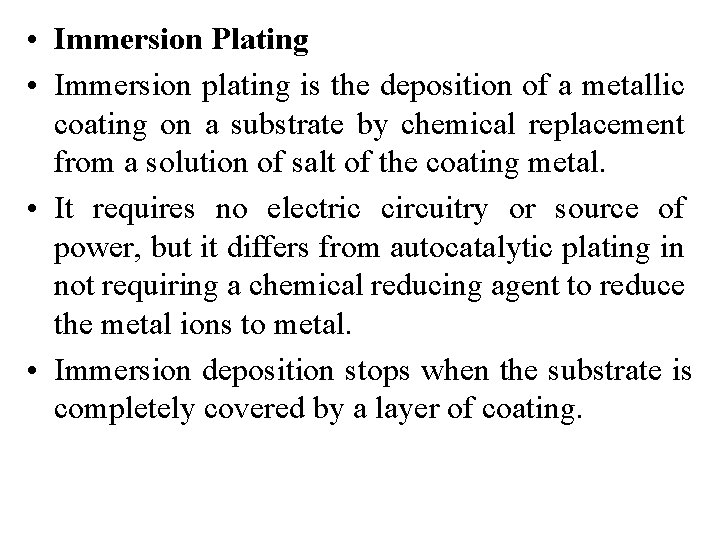
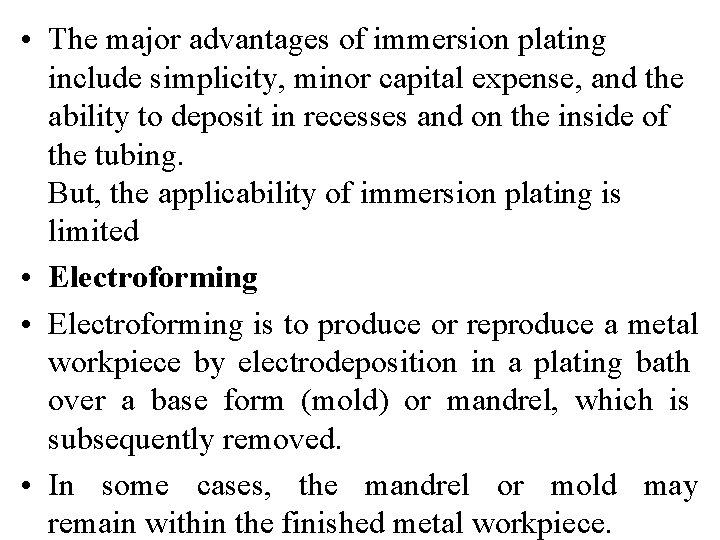
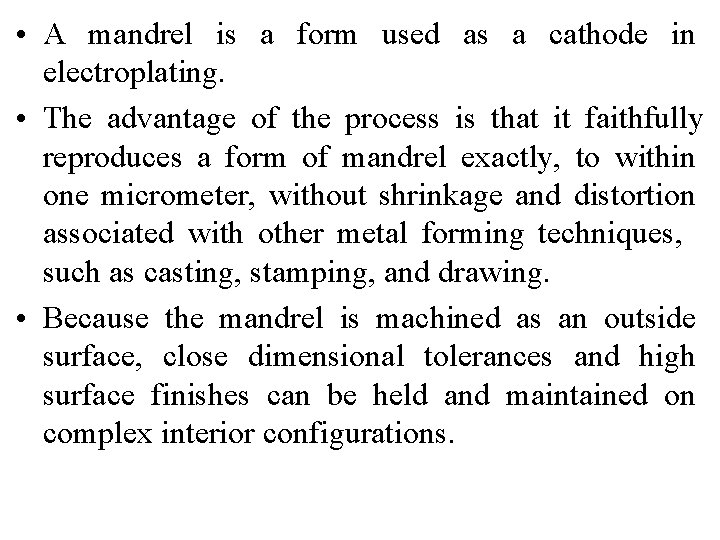
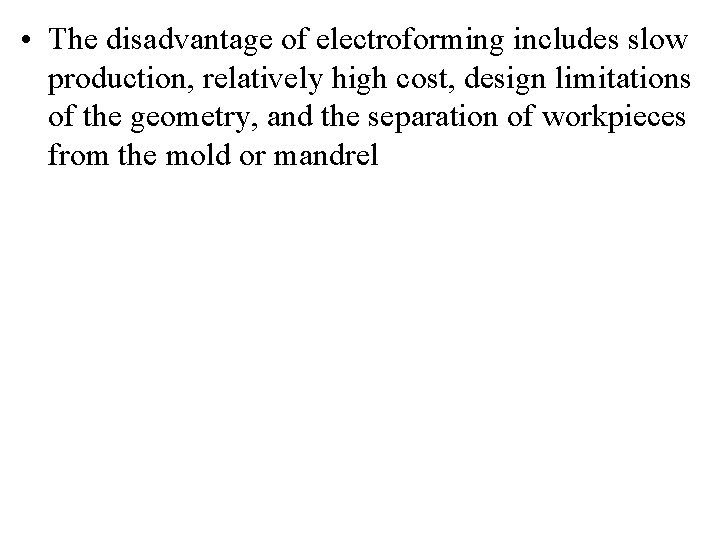
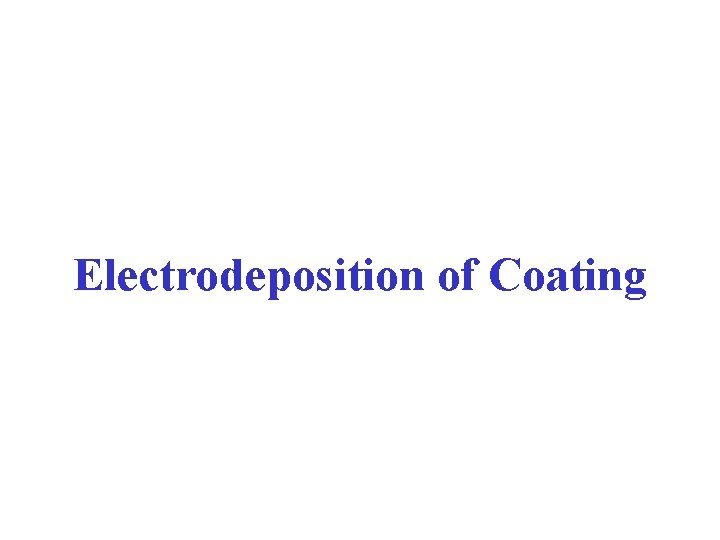
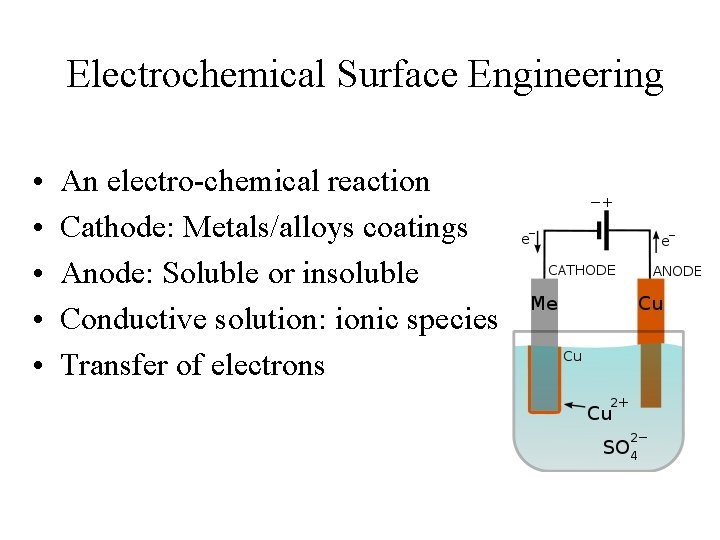
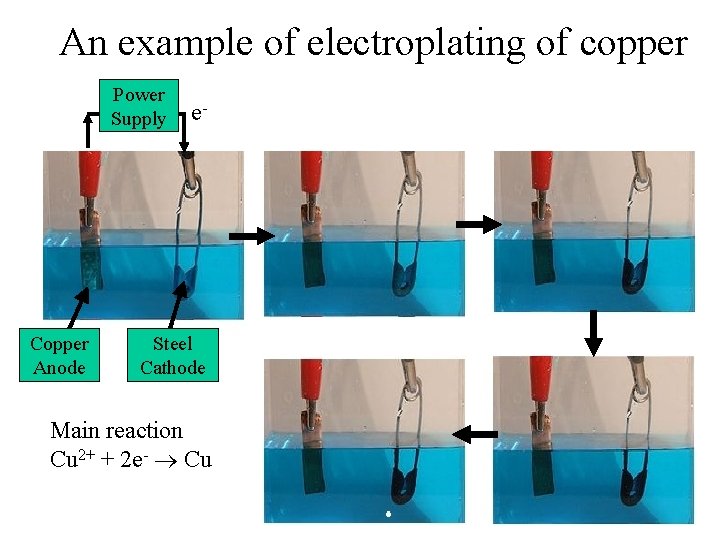
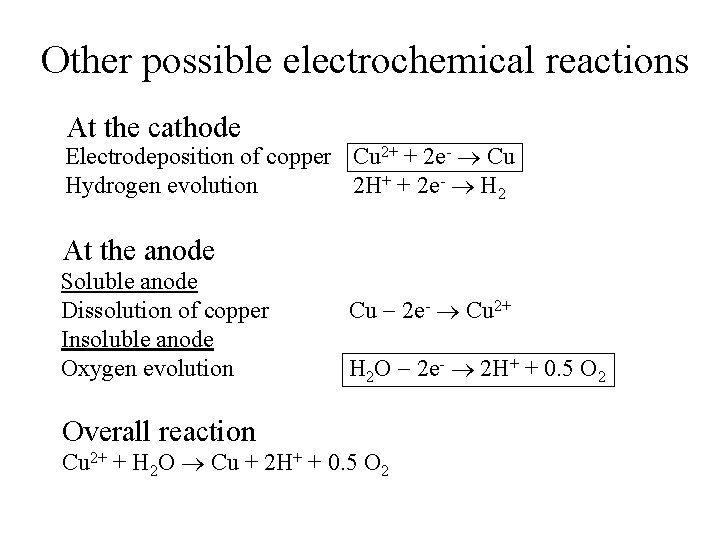
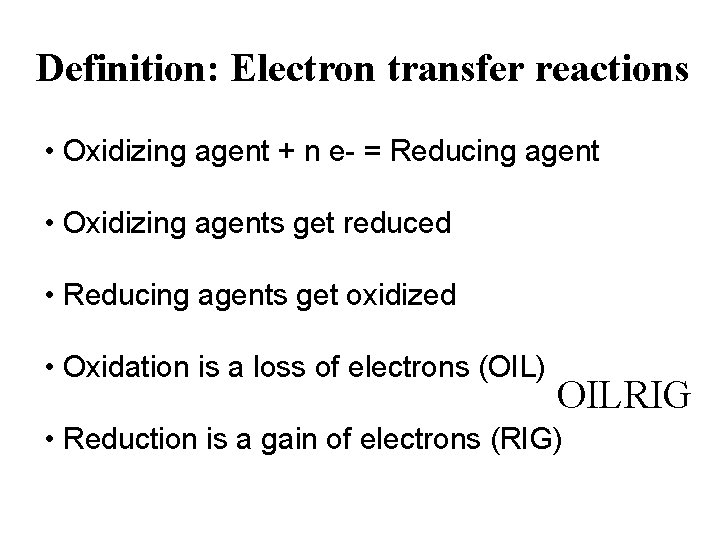
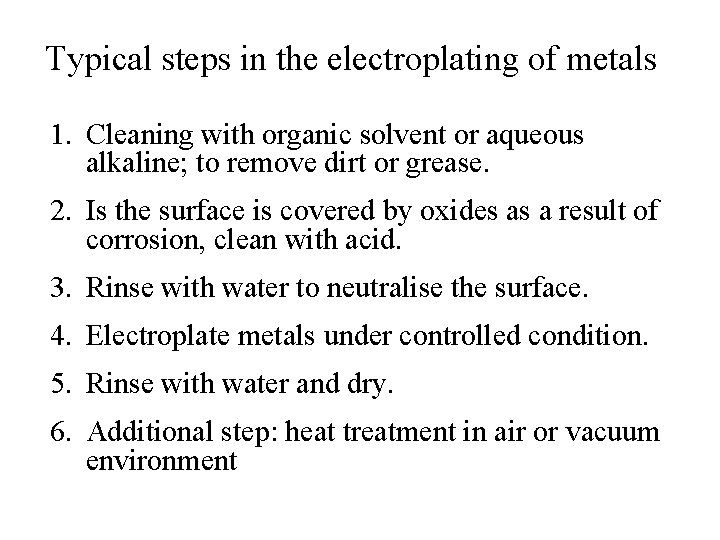
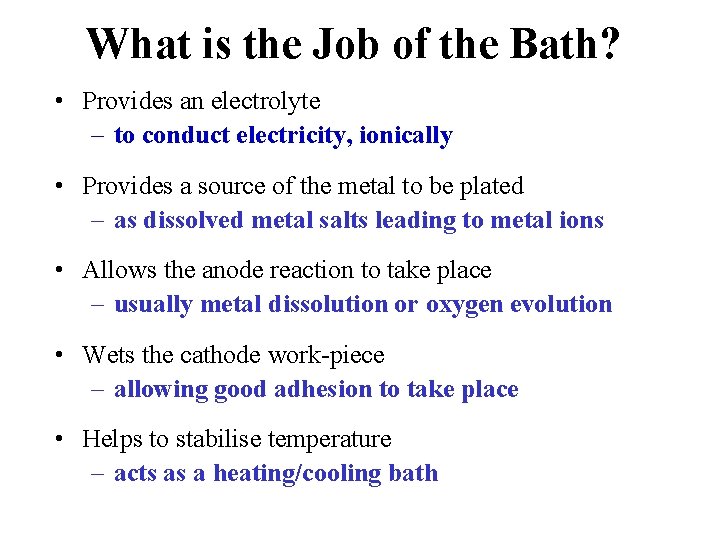
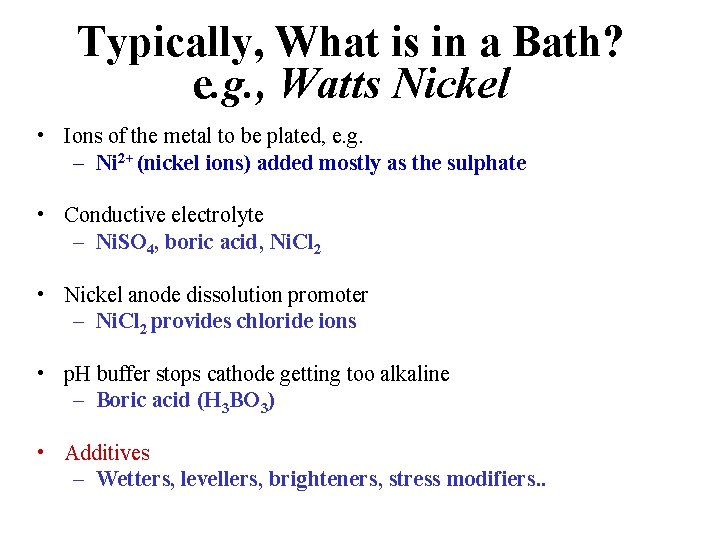
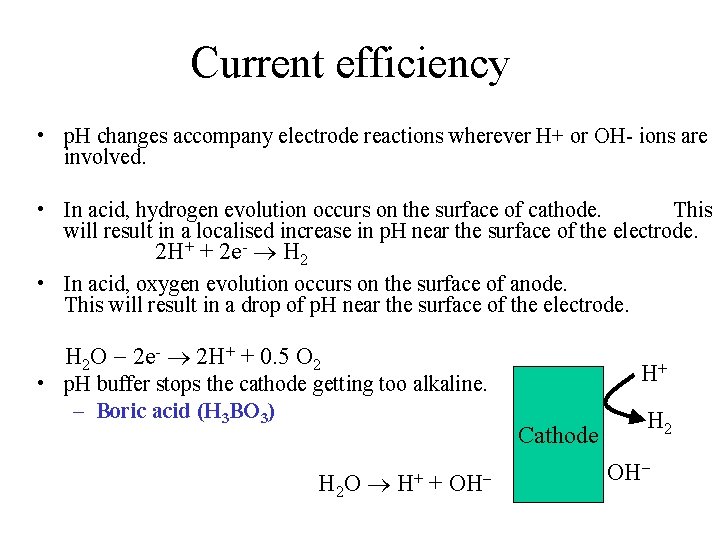
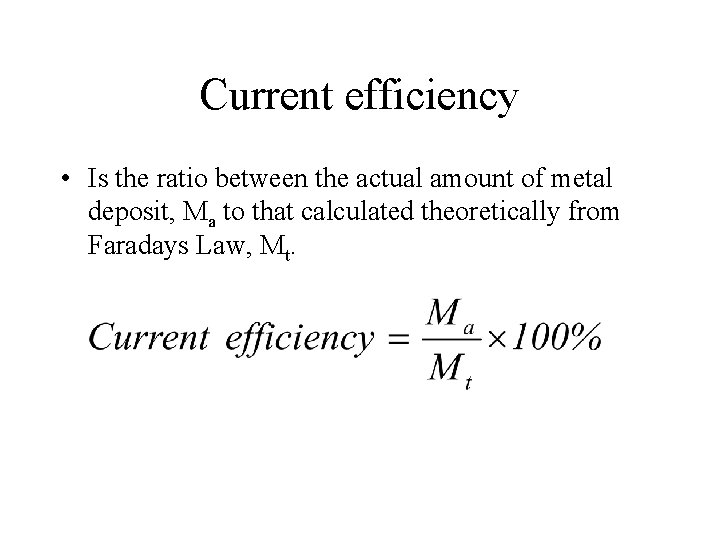
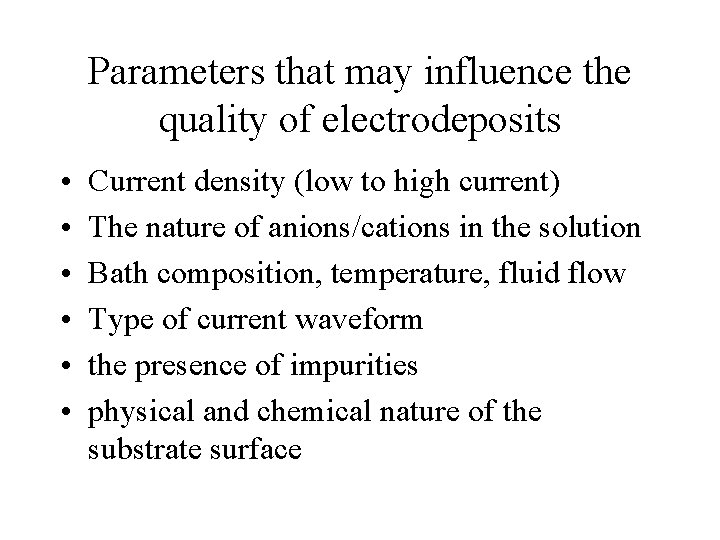
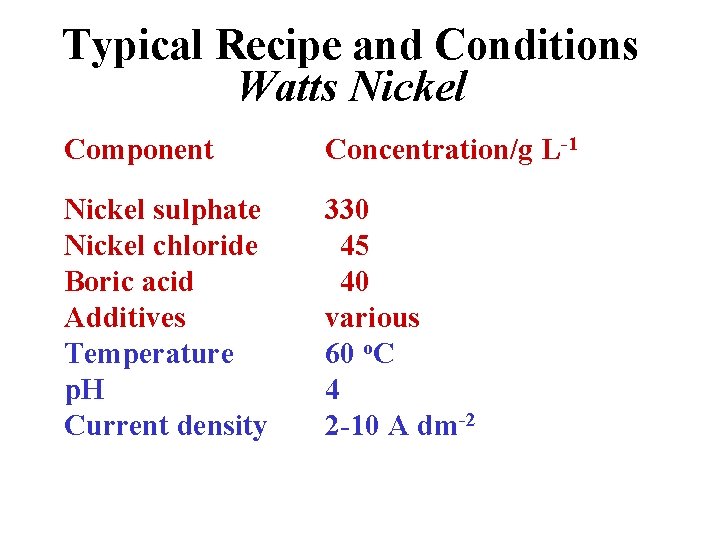
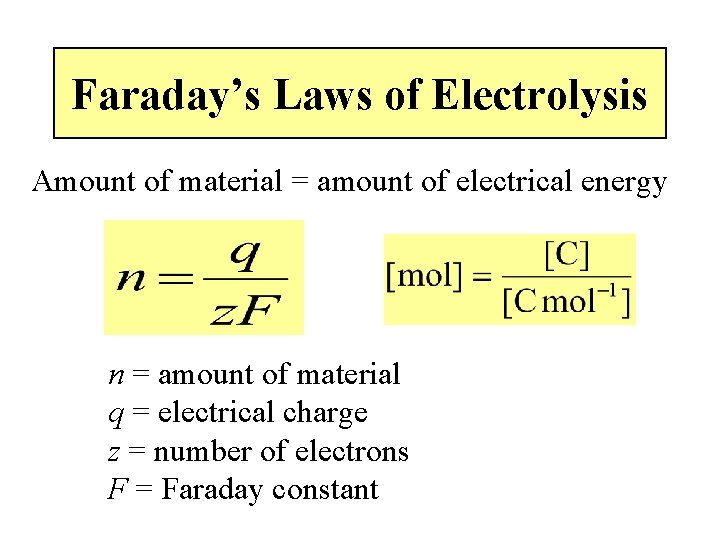
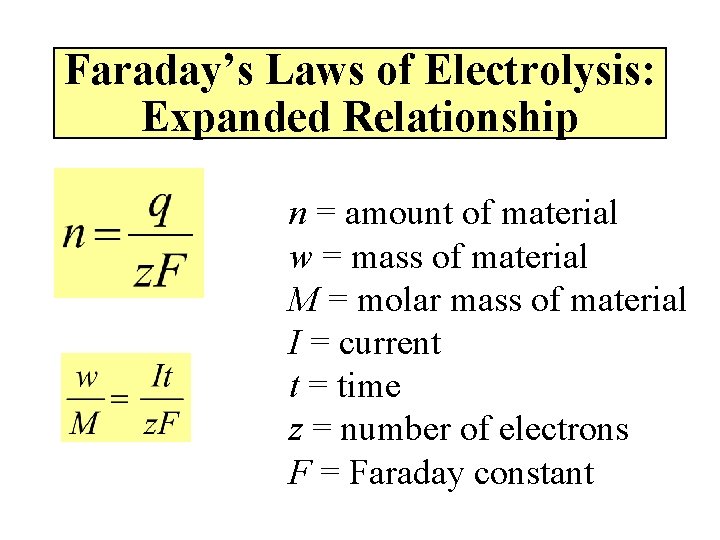
![Current, Current density, Surface area j = current density [m. A cm-2] I = Current, Current density, Surface area j = current density [m. A cm-2] I =](https://slidetodoc.com/presentation_image_h2/78f3b1ea4895bc2c5935e6be093fcd7a/image-104.jpg)
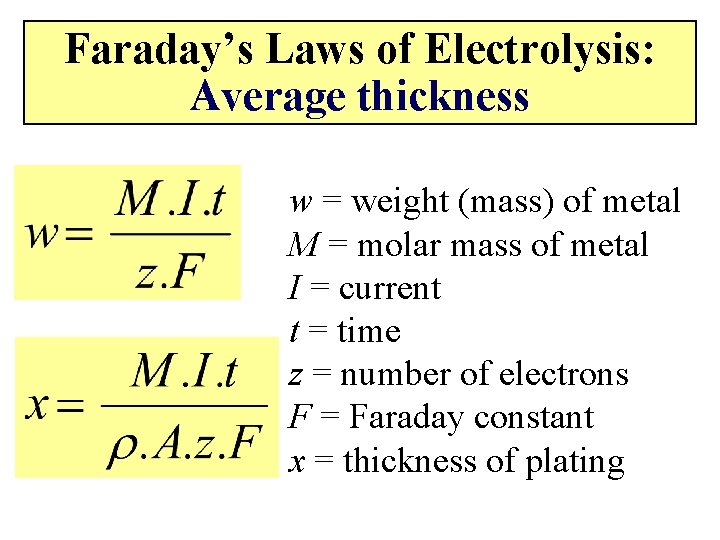
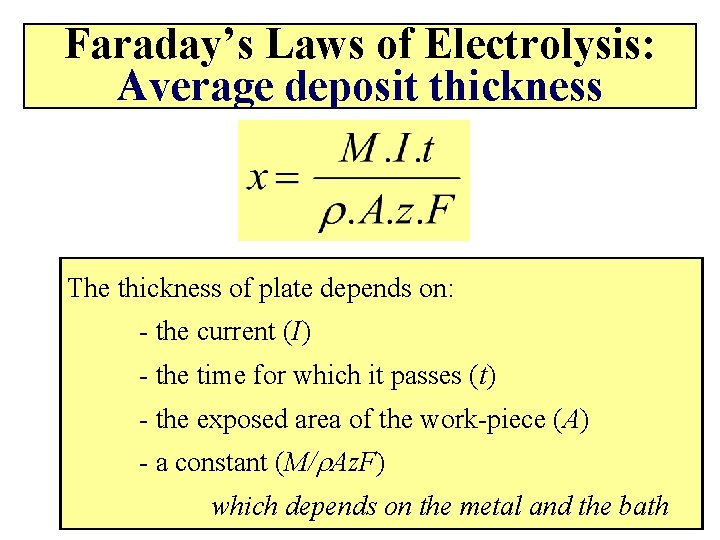
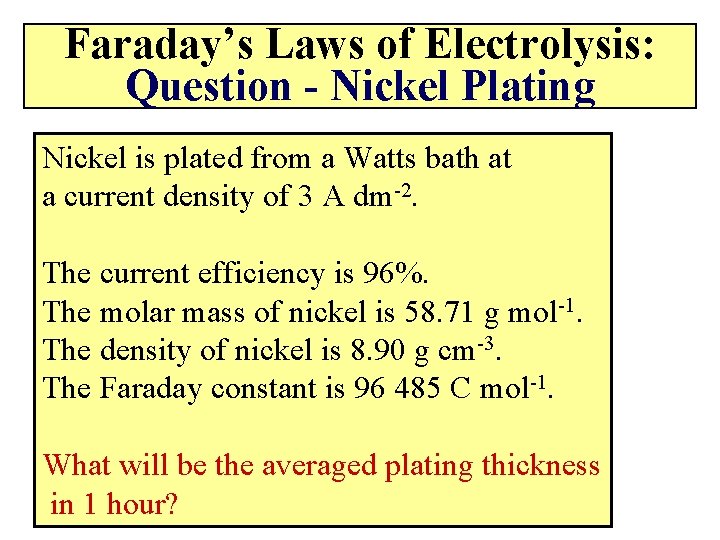
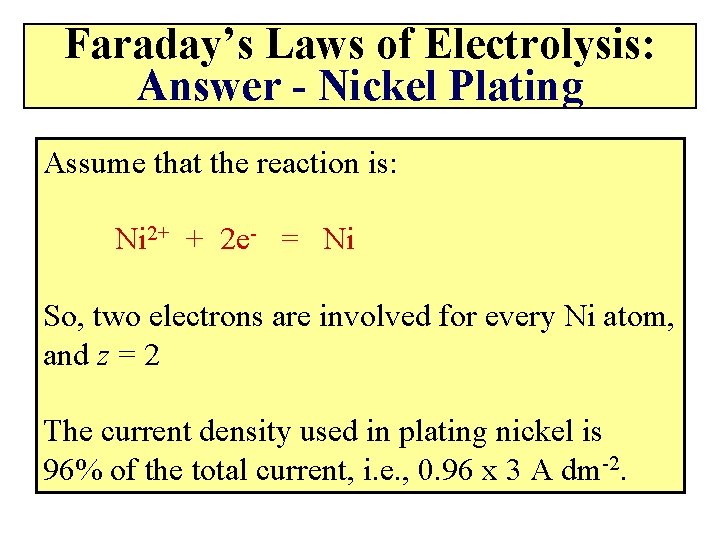
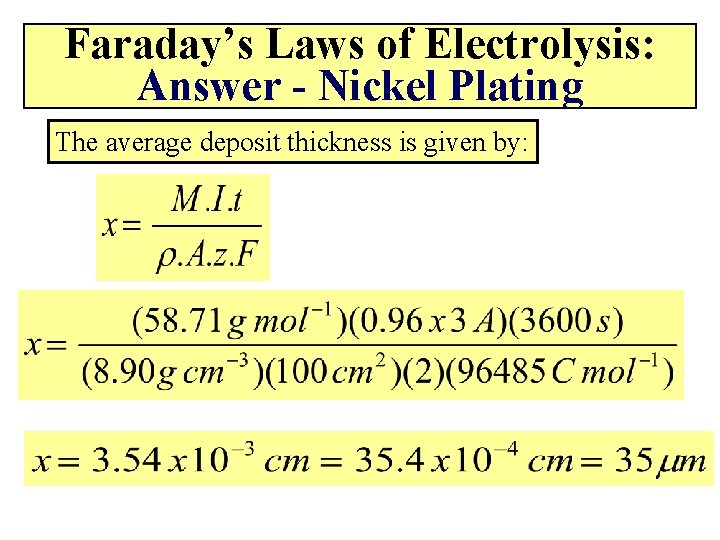
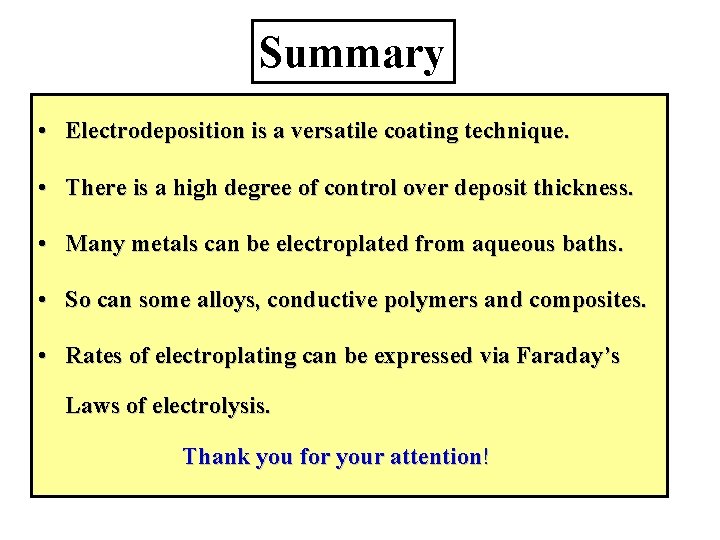
- Slides: 110
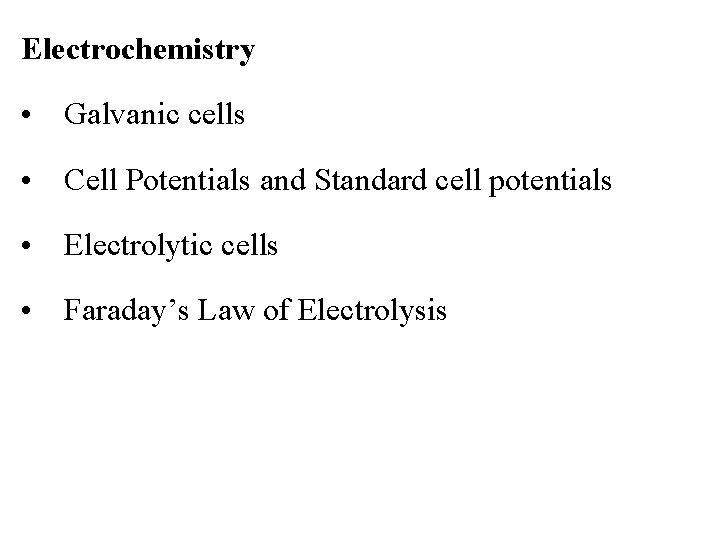
Electrochemistry • Galvanic cells • Cell Potentials and Standard cell potentials • Electrolytic cells • Faraday’s Law of Electrolysis
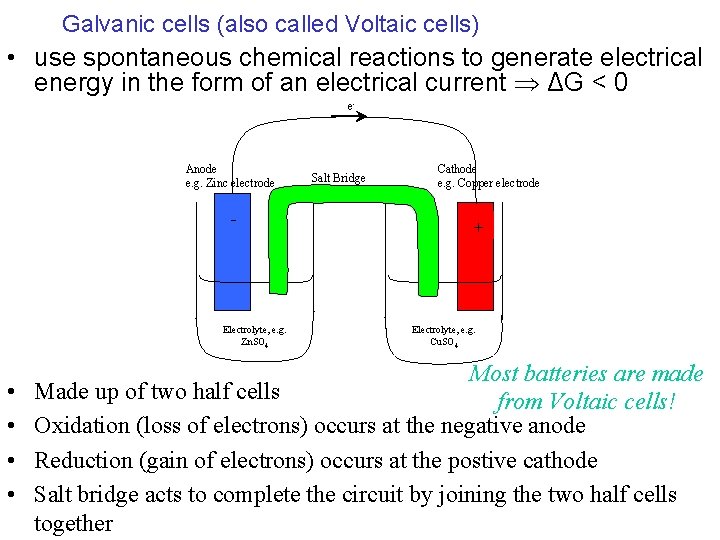
Galvanic cells (also called Voltaic cells) • use spontaneous chemical reactions to generate electrical energy in the form of an electrical current ΔG < 0 e- Anode e. g. Zinc electrode - Electrolyte, e. g. Zn. SO 4 • • Salt Bridge Cathode e. g. Copper electrode + Electrolyte, e. g. Cu. SO 4 Most batteries are made Made up of two half cells from Voltaic cells! Oxidation (loss of electrons) occurs at the negative anode Reduction (gain of electrons) occurs at the postive cathode Salt bridge acts to complete the circuit by joining the two half cells together
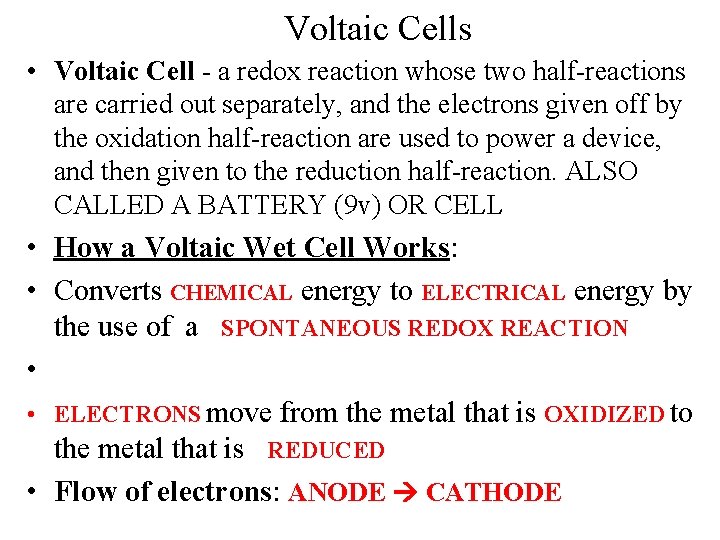
Voltaic Cells • Voltaic Cell - a redox reaction whose two half-reactions are carried out separately, and the electrons given off by the oxidation half-reaction are used to power a device, and then given to the reduction half-reaction. ALSO CALLED A BATTERY (9 v) OR CELL • How a Voltaic Wet Cell Works: • Converts CHEMICAL energy to ELECTRICAL energy by the use of a SPONTANEOUS REDOX REACTION • • ELECTRONS move from the metal that is OXIDIZED to the metal that is REDUCED • Flow of electrons: ANODE CATHODE
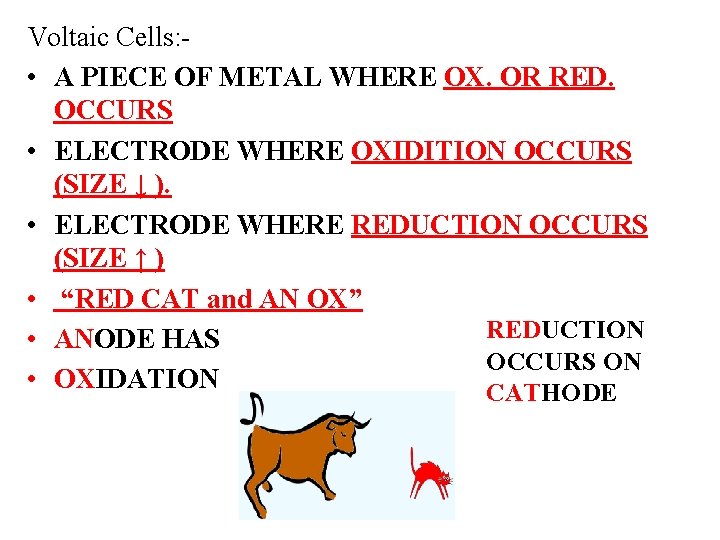
Voltaic Cells: • A PIECE OF METAL WHERE OX. OR RED. OCCURS • ELECTRODE WHERE OXIDITION OCCURS (SIZE ↓ ). • ELECTRODE WHERE REDUCTION OCCURS (SIZE ↑ ) • “RED CAT and AN OX” REDUCTION • ANODE HAS OCCURS ON • OXIDATION CATHODE
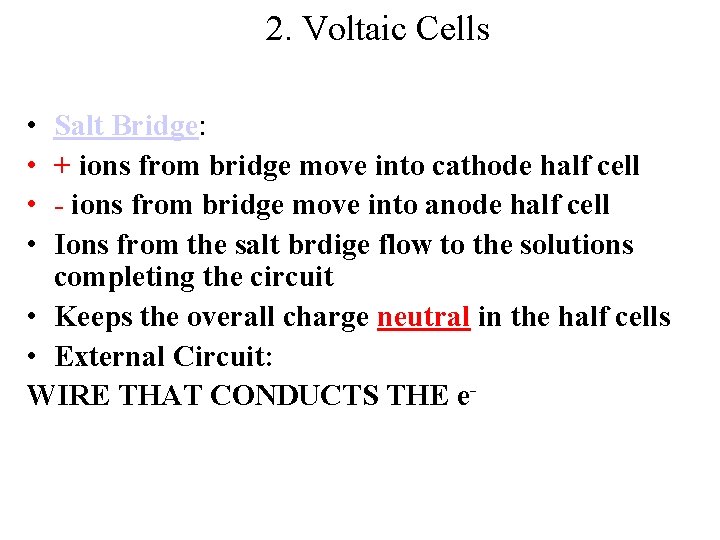
2. Voltaic Cells • • Salt Bridge: + ions from bridge move into cathode half cell - ions from bridge move into anode half cell Ions from the salt brdige flow to the solutions completing the circuit • Keeps the overall charge neutral in the half cells • External Circuit: WIRE THAT CONDUCTS THE e-
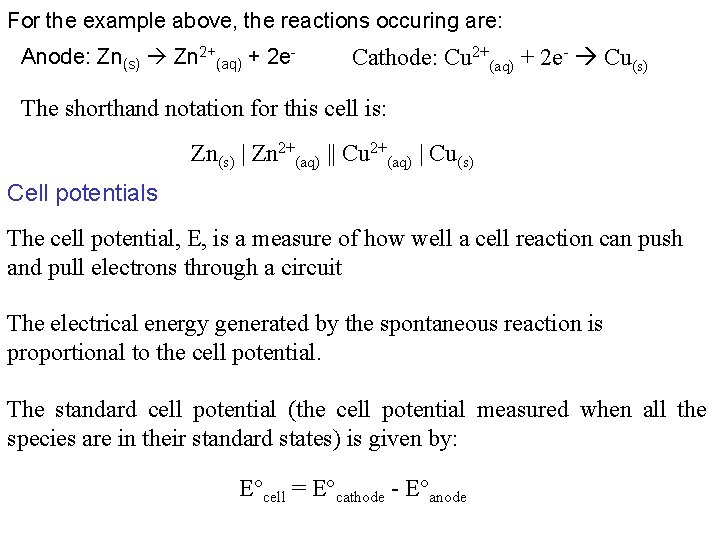
For the example above, the reactions occuring are: Anode: Zn(s) Zn 2+(aq) + 2 e- Cathode: Cu 2+(aq) + 2 e- Cu(s) The shorthand notation for this cell is: Zn(s) | Zn 2+(aq) || Cu 2+(aq) | Cu(s) Cell potentials The cell potential, E, is a measure of how well a cell reaction can push and pull electrons through a circuit The electrical energy generated by the spontaneous reaction is proportional to the cell potential. The standard cell potential (the cell potential measured when all the species are in their standard states) is given by: E°cell = E°cathode - E°anode
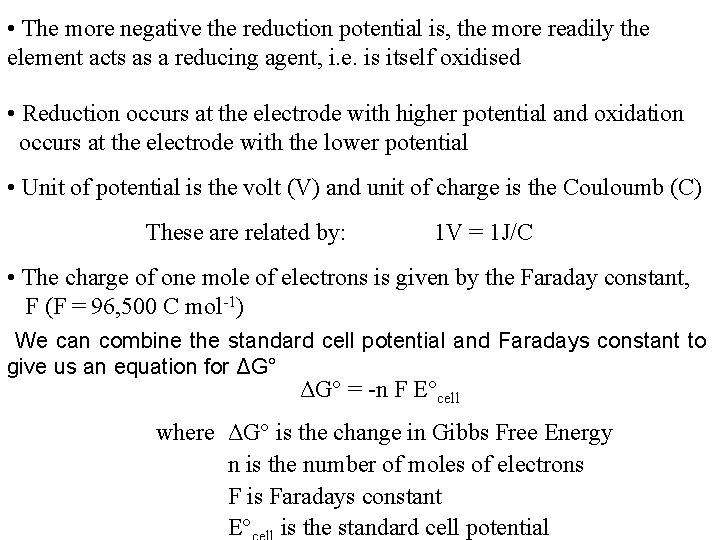
• The more negative the reduction potential is, the more readily the element acts as a reducing agent, i. e. is itself oxidised • Reduction occurs at the electrode with higher potential and oxidation occurs at the electrode with the lower potential • Unit of potential is the volt (V) and unit of charge is the Couloumb (C) These are related by: 1 V = 1 J/C • The charge of one mole of electrons is given by the Faraday constant, F (F = 96, 500 C mol-1) We can combine the standard cell potential and Faradays constant to give us an equation for ΔG° = -n F E°cell where ΔG° is the change in Gibbs Free Energy n is the number of moles of electrons F is Faradays constant E° is the standard cell potential
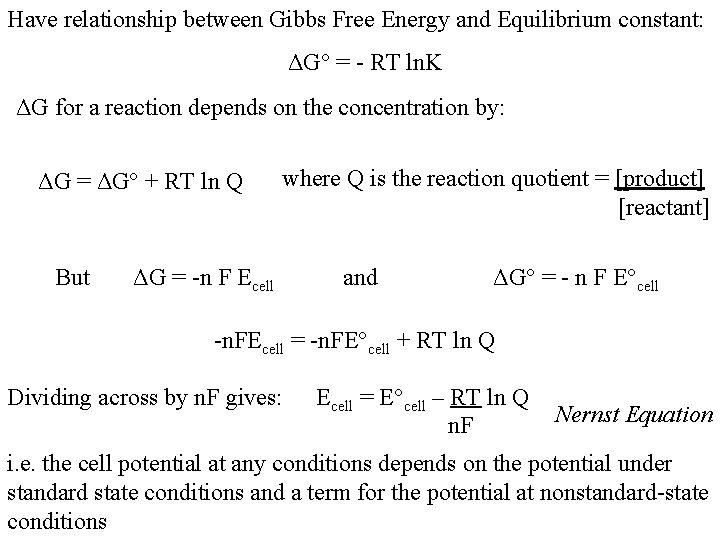
Have relationship between Gibbs Free Energy and Equilibrium constant: ΔG° = - RT ln. K ΔG for a reaction depends on the concentration by: ΔG = ΔG° + RT ln Q But where Q is the reaction quotient = [product] [reactant] ΔG = -n F Ecell and ΔG° = - n F E°cell -n. FEcell = -n. FE°cell + RT ln Q Dividing across by n. F gives: Ecell = E°cell – RT ln Q n. F Nernst Equation i. e. the cell potential at any conditions depends on the potential under standard state conditions and a term for the potential at nonstandard-state conditions
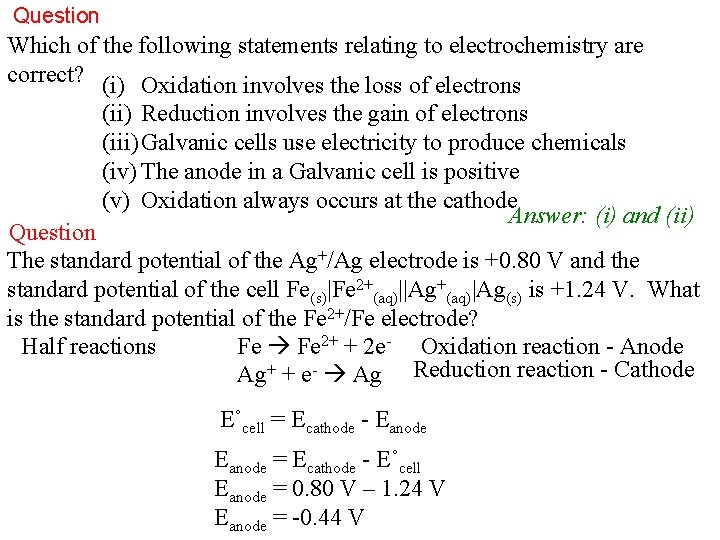
Question Which of the following statements relating to electrochemistry are correct? (i) Oxidation involves the loss of electrons (ii) Reduction involves the gain of electrons (iii) Galvanic cells use electricity to produce chemicals (iv) The anode in a Galvanic cell is positive (v) Oxidation always occurs at the cathode Answer: (i) and (ii) Question The standard potential of the Ag+/Ag electrode is +0. 80 V and the standard potential of the cell Fe(s)|Fe 2+(aq)||Ag+(aq)|Ag(s) is +1. 24 V. What is the standard potential of the Fe 2+/Fe electrode? Half reactions Fe 2+ + 2 e- Oxidation reaction - Anode Ag+ + e- Ag Reduction reaction - Cathode E˚cell = Ecathode - Eanode = Ecathode - E˚cell Eanode = 0. 80 V – 1. 24 V Eanode = -0. 44 V
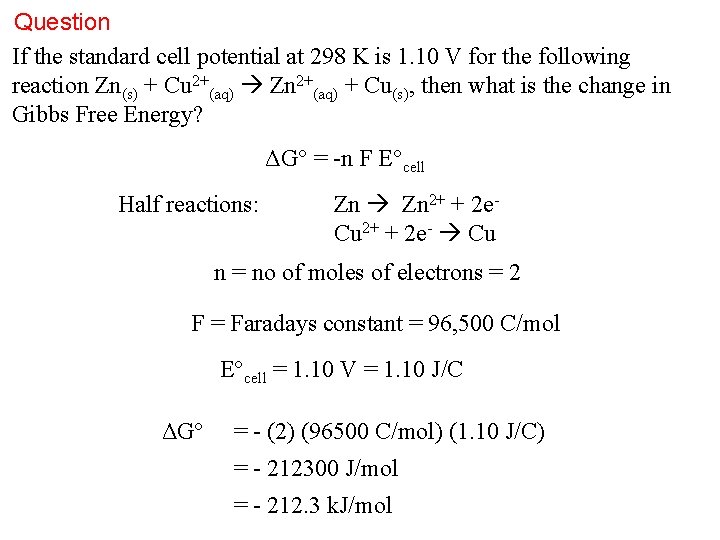
Question If the standard cell potential at 298 K is 1. 10 V for the following reaction Zn(s) + Cu 2+(aq) Zn 2+(aq) + Cu(s), then what is the change in Gibbs Free Energy? ΔG° = -n F E°cell Half reactions: Zn 2+ + 2 e. Cu 2+ + 2 e- Cu n = no of moles of electrons = 2 F = Faradays constant = 96, 500 C/mol E°cell = 1. 10 V = 1. 10 J/C ΔG° = - (2) (96500 C/mol) (1. 10 J/C) = - 212300 J/mol = - 212. 3 k. J/mol
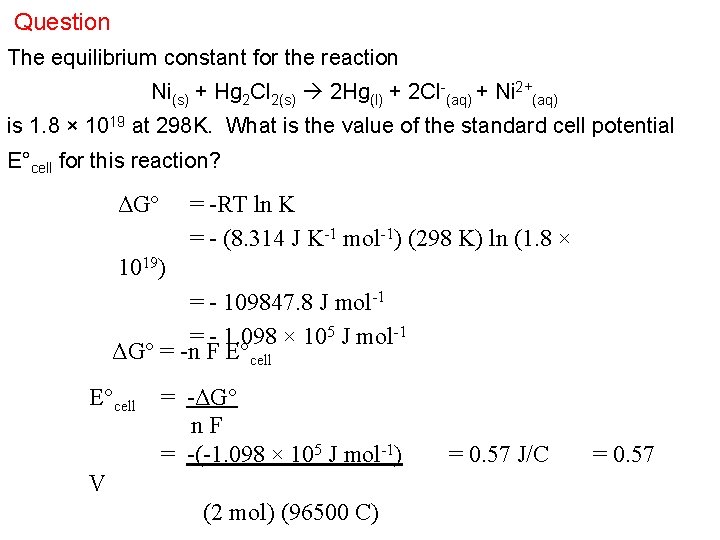
Question The equilibrium constant for the reaction Ni(s) + Hg 2 Cl 2(s) 2 Hg(l) + 2 Cl-(aq) + Ni 2+(aq) is 1. 8 × 1019 at 298 K. What is the value of the standard cell potential E°cell for this reaction? ΔG° = -RT ln K = - (8. 314 J K-1 mol-1) (298 K) ln (1. 8 × 1019) = - 109847. 8 J mol-1 = - 1. 098 × 105 J mol-1 ΔG° = -n F E°cell = -ΔG° n. F = -(-1. 098 × 105 J mol-1) V (2 mol) (96500 C) = 0. 57 J/C = 0. 57
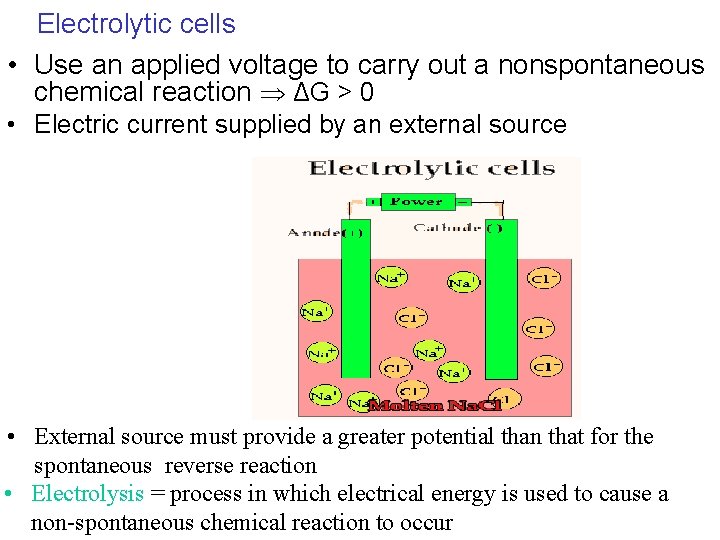
Electrolytic cells • Use an applied voltage to carry out a nonspontaneous chemical reaction ΔG > 0 • Electric current supplied by an external source • External source must provide a greater potential than that for the spontaneous reverse reaction • Electrolysis = process in which electrical energy is used to cause a non-spontaneous chemical reaction to occur
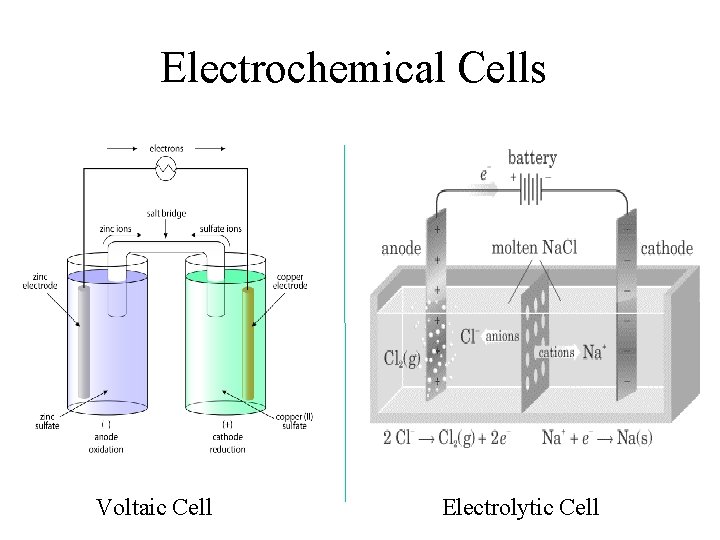
Electrochemical Cells Voltaic Cell Electrolytic Cell
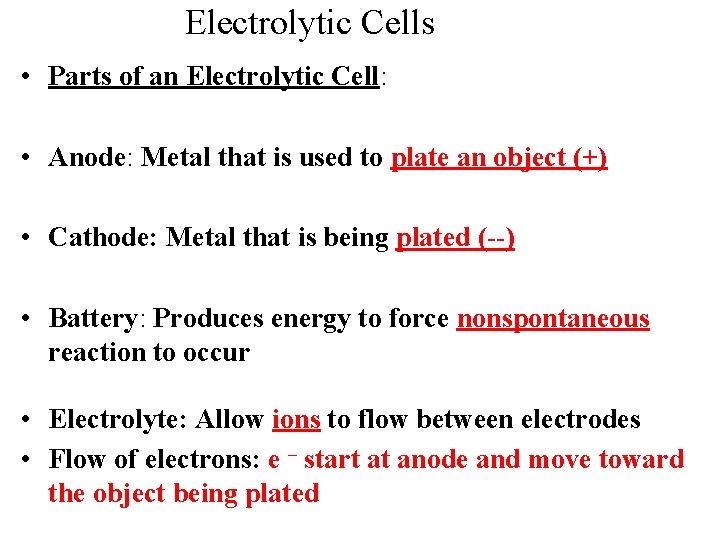
Electrolytic Cells • Parts of an Electrolytic Cell: • Anode: Metal that is used to plate an object (+) • Cathode: Metal that is being plated (--) • Battery: Produces energy to force nonspontaneous reaction to occur • Electrolyte: Allow ions to flow between electrodes • Flow of electrons: e – start at anode and move toward the object being plated
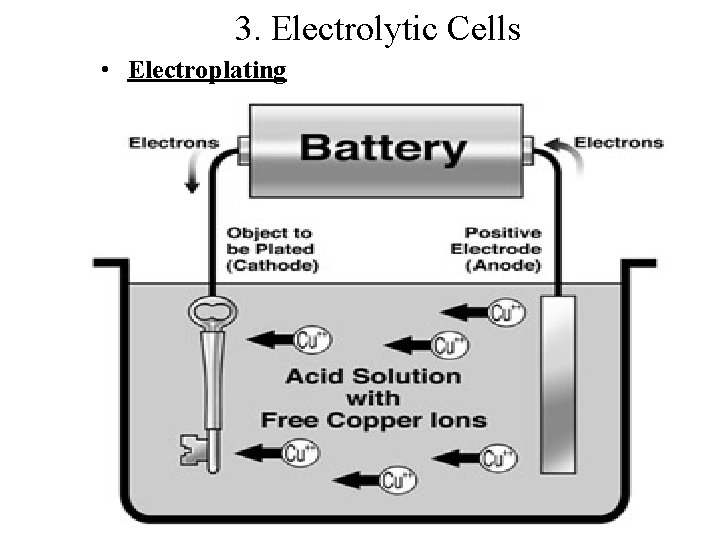
3. Electrolytic Cells • Electroplating
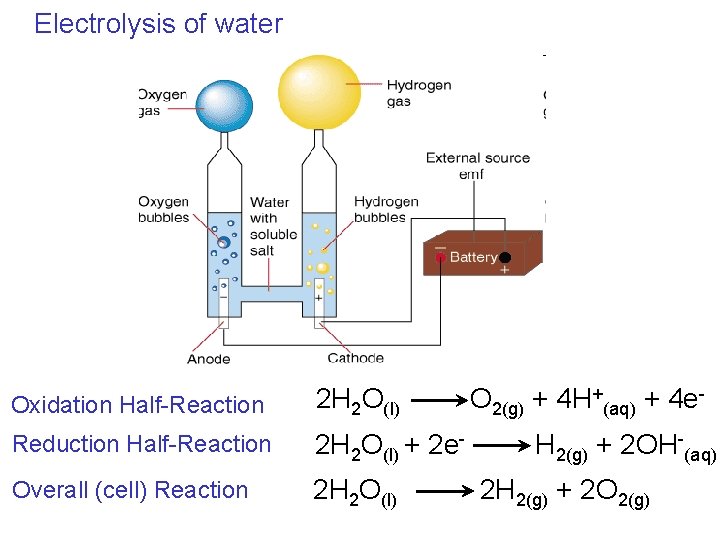
Electrolysis of water Oxidation Half-Reaction 2 H 2 O(l) Reduction Half-Reaction 2 H 2 O(l) + 2 e- Overall (cell) Reaction 2 H 2 O(l) O 2(g) + 4 H+(aq) + 4 e. H 2(g) + 2 OH-(aq) 2 H 2(g) + 2 O 2(g)
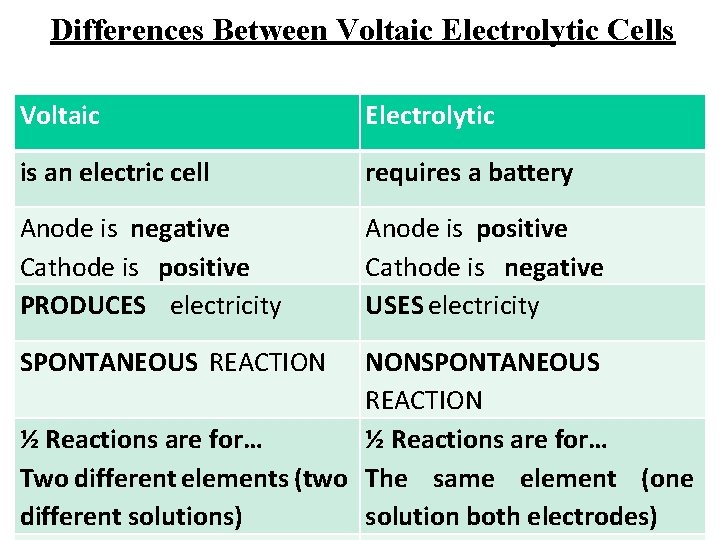
Differences Between Voltaic Electrolytic Cells Voltaic Electrolytic is an electric cell requires a battery Anode is negative Cathode is positive PRODUCES electricity Anode is positive Cathode is negative USES electricity SPONTANEOUS REACTION NONSPONTANEOUS REACTION ½ Reactions are for… Two different elements (two The same element (one different solutions) solution both electrodes)
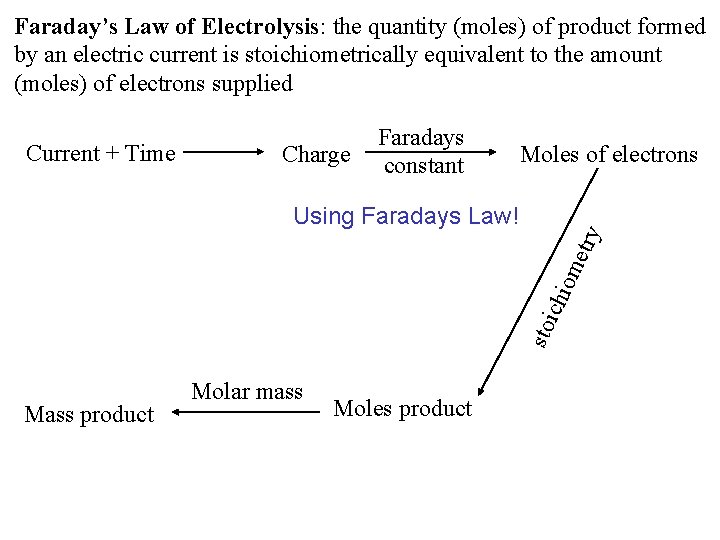
Faraday’s Law of Electrolysis: the quantity (moles) of product formed by an electric current is stoichiometrically equivalent to the amount (moles) of electrons supplied Current + Time Charge Faradays constant Moles of electrons sto ich iom etry Using Faradays Law! Mass product Molar mass Moles product
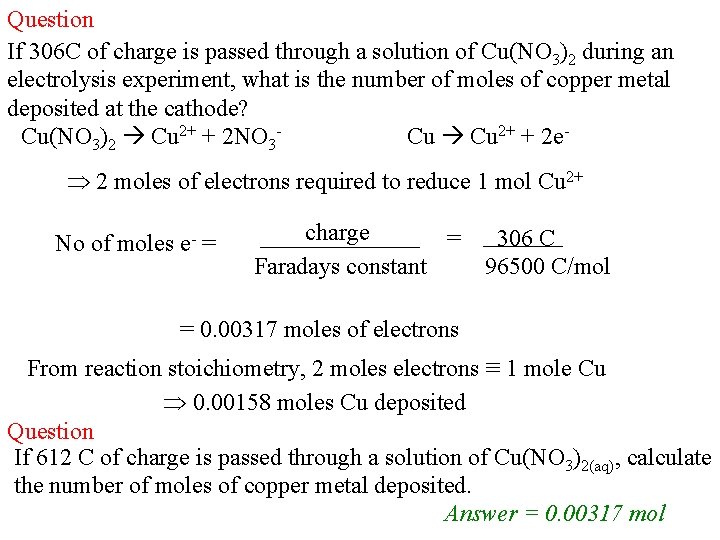
Question If 306 C of charge is passed through a solution of Cu(NO 3)2 during an electrolysis experiment, what is the number of moles of copper metal deposited at the cathode? Cu(NO 3)2 Cu 2+ + 2 NO 3 Cu Cu 2+ + 2 e 2 moles of electrons required to reduce 1 mol Cu 2+ No of moles e- = charge = Faradays constant 306 C 96500 C/mol = 0. 00317 moles of electrons From reaction stoichiometry, 2 moles electrons ≡ 1 mole Cu 0. 00158 moles Cu deposited Question If 612 C of charge is passed through a solution of Cu(NO 3)2(aq), calculate the number of moles of copper metal deposited. Answer = 0. 00317 mol
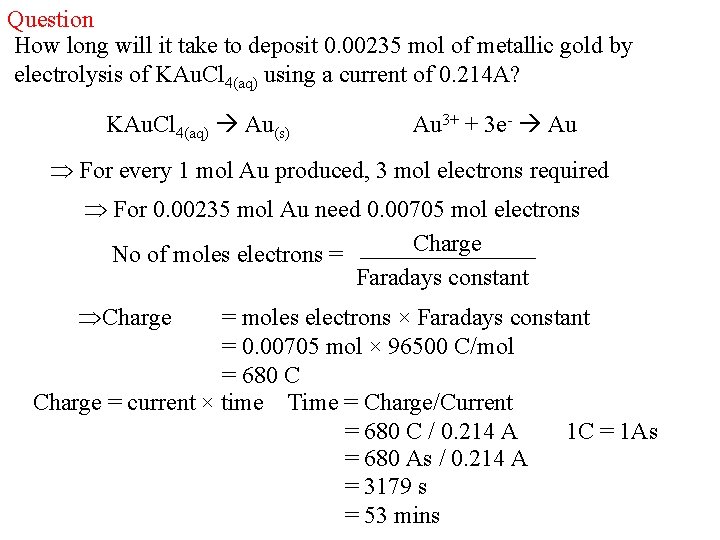
Question How long will it take to deposit 0. 00235 mol of metallic gold by electrolysis of KAu. Cl 4(aq) using a current of 0. 214 A? KAu. Cl 4(aq) Au(s) Au 3+ + 3 e- Au For every 1 mol Au produced, 3 mol electrons required For 0. 00235 mol Au need 0. 00705 mol electrons Charge No of moles electrons = Faradays constant Charge = moles electrons × Faradays constant = 0. 00705 mol × 96500 C/mol = 680 C Charge = current × time Time = Charge/Current 1 C = 1 As = 680 C / 0. 214 A = 680 As / 0. 214 A = 3179 s = 53 mins
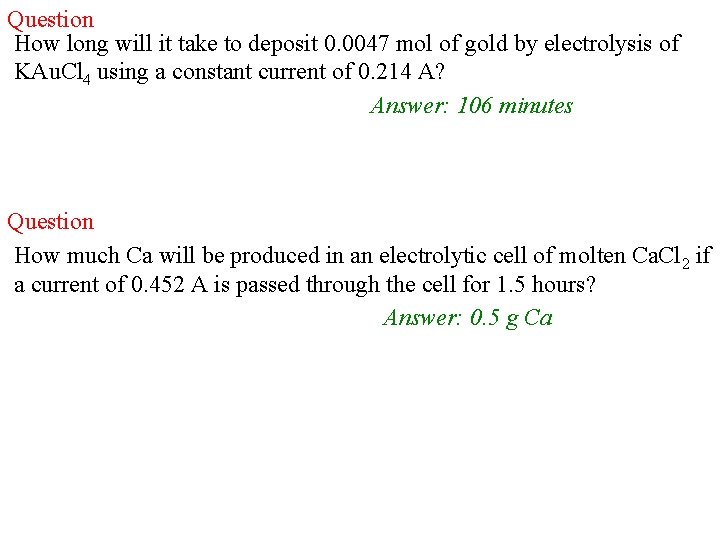
Question How long will it take to deposit 0. 0047 mol of gold by electrolysis of KAu. Cl 4 using a constant current of 0. 214 A? Answer: 106 minutes Question How much Ca will be produced in an electrolytic cell of molten Ca. Cl 2 if a current of 0. 452 A is passed through the cell for 1. 5 hours? Answer: 0. 5 g Ca
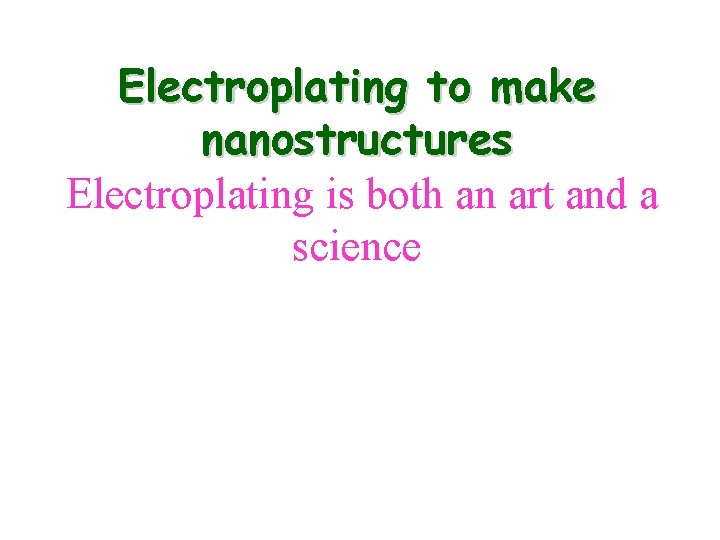
Electroplating to make nanostructures Electroplating is both an art and a science
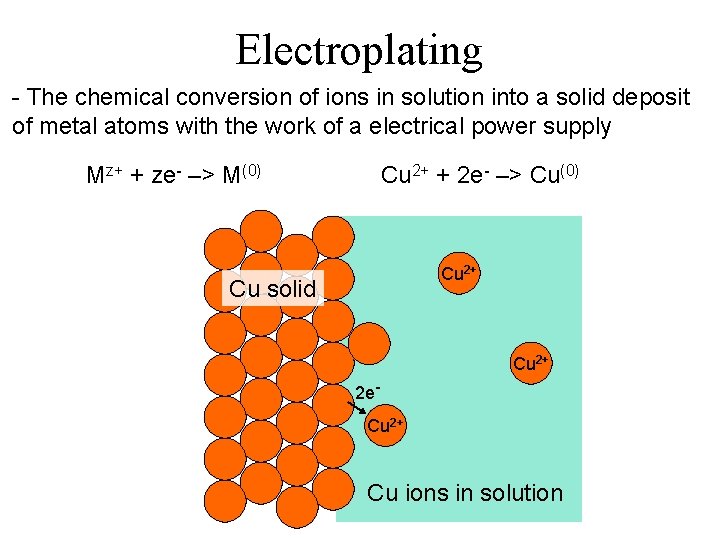
Electroplating - The chemical conversion of ions in solution into a solid deposit of metal atoms with the work of a electrical power supply Mz+ + ze- –> M(0) Cu 2+ + 2 e- –> Cu(0) Cu 2+ Cu solid Cu 2+ 2 e. Cu 2+ Cu ions in solution
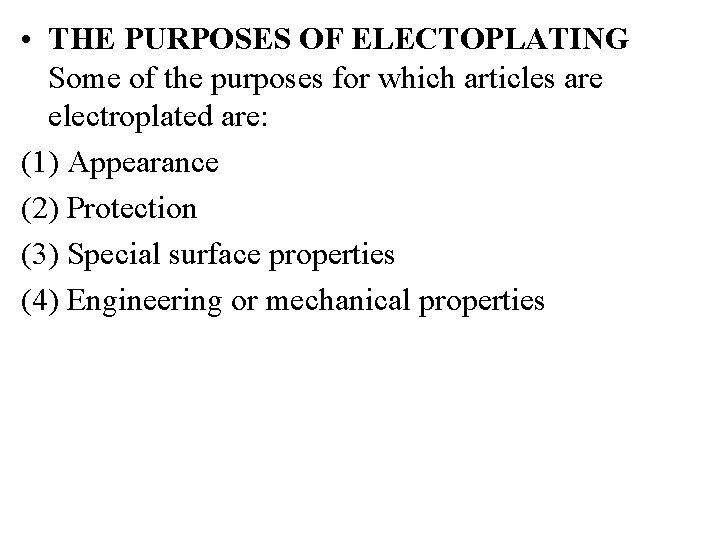
• THE PURPOSES OF ELECTOPLATING Some of the purposes for which articles are electroplated are: (1) Appearance (2) Protection (3) Special surface properties (4) Engineering or mechanical properties
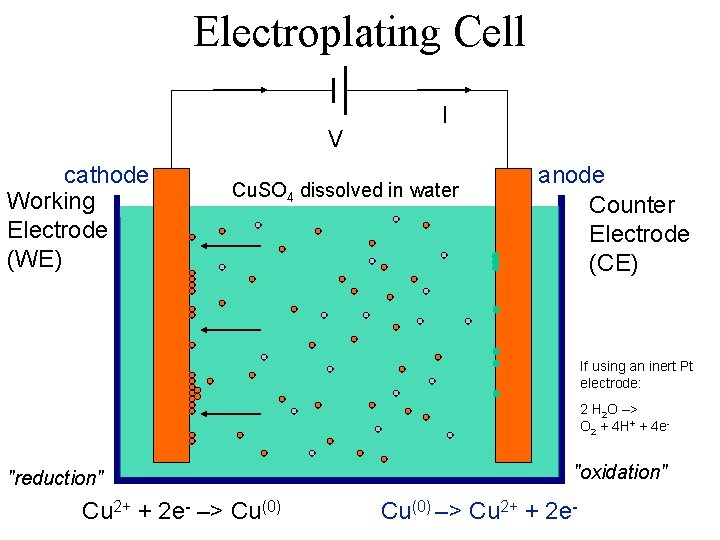
Electroplating Cell V cathode Working Electrode (WE) I Cu. SO 4 dissolved in water anode Counter Electrode (CE) If using an inert Pt electrode: 2 H 2 O –> O 2 + 4 H+ + 4 e- "reduction" Cu 2+ + 2 e- –> Cu(0) "oxidation" Cu(0) –> Cu 2+ + 2 e-
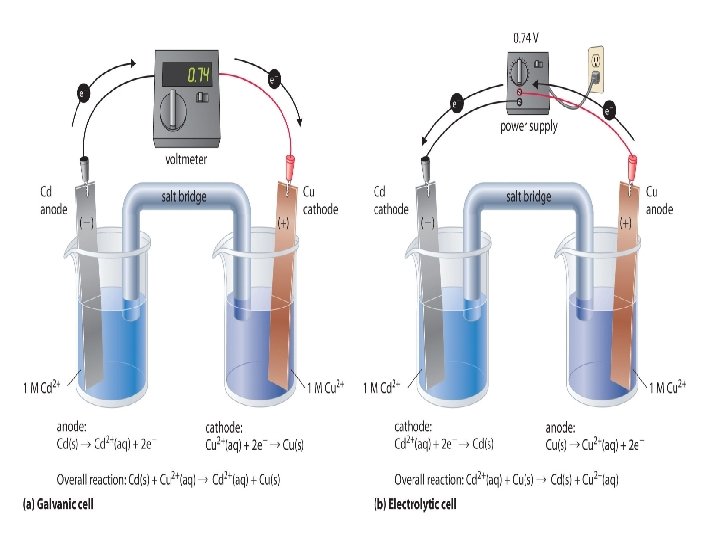
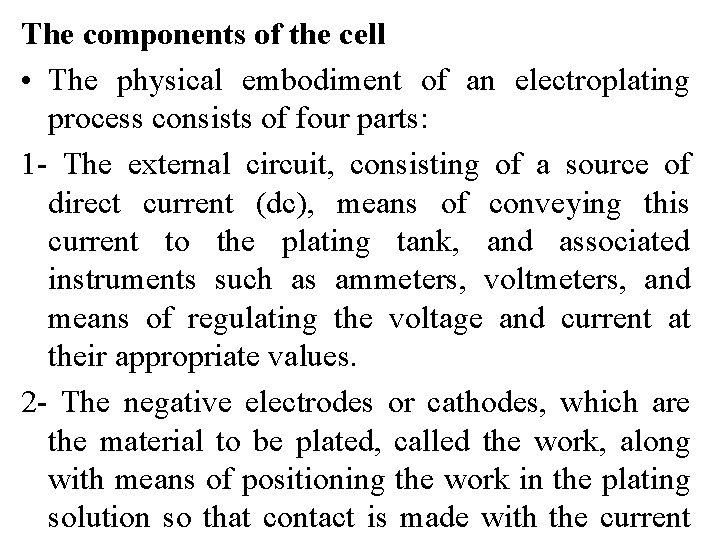
The components of the cell • The physical embodiment of an electroplating process consists of four parts: 1 - The external circuit, consisting of a source of direct current (dc), means of conveying this current to the plating tank, and associated instruments such as ammeters, voltmeters, and means of regulating the voltage and current at their appropriate values. 2 - The negative electrodes or cathodes, which are the material to be plated, called the work, along with means of positioning the work in the plating solution so that contact is made with the current
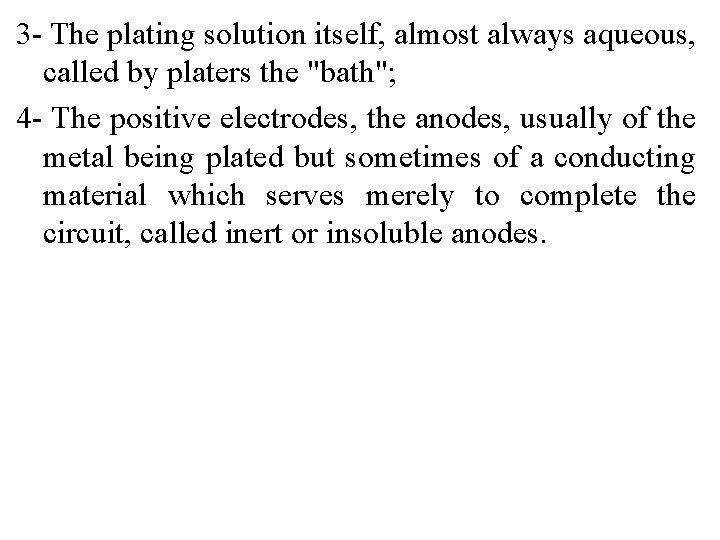
3 - The plating solution itself, almost always aqueous, called by platers the "bath"; 4 - The positive electrodes, the anodes, usually of the metal being plated but sometimes of a conducting material which serves merely to complete the circuit, called inert or insoluble anodes.
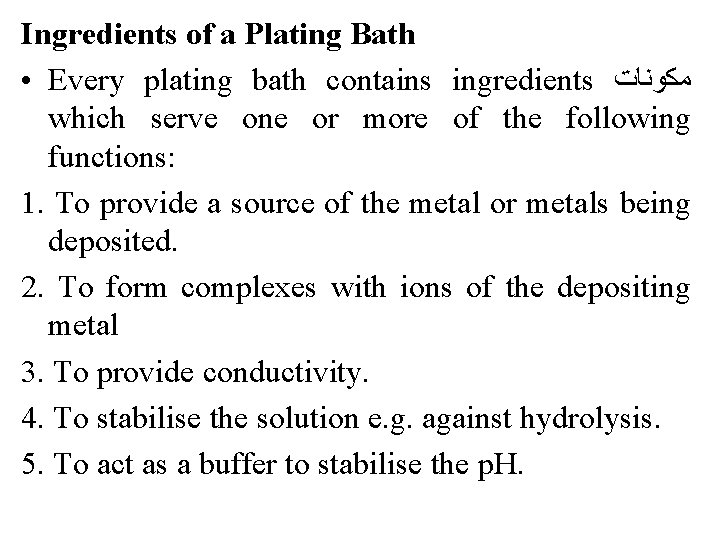
Ingredients of a Plating Bath • Every plating bath contains ingredients ﻣﻜﻮﻧﺎﺕ which serve one or more of the following functions: 1. To provide a source of the metal or metals being deposited. 2. To form complexes with ions of the depositing metal 3. To provide conductivity. 4. To stabilise the solution e. g. against hydrolysis. 5. To act as a buffer to stabilise the p. H.
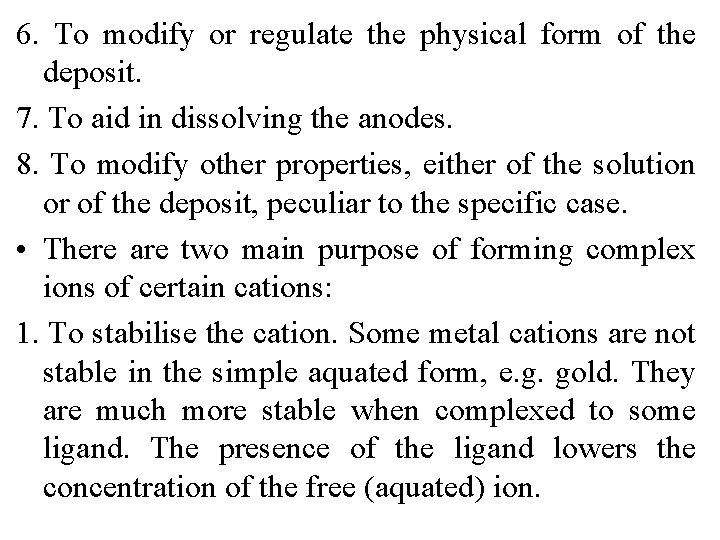
6. To modify or regulate the physical form of the deposit. 7. To aid in dissolving the anodes. 8. To modify other properties, either of the solution or of the deposit, peculiar to the specific case. • There are two main purpose of forming complex ions of certain cations: 1. To stabilise the cation. Some metal cations are not stable in the simple aquated form, e. g. gold. They are much more stable when complexed to some ligand. The presence of the ligand lowers the concentration of the free (aquated) ion.
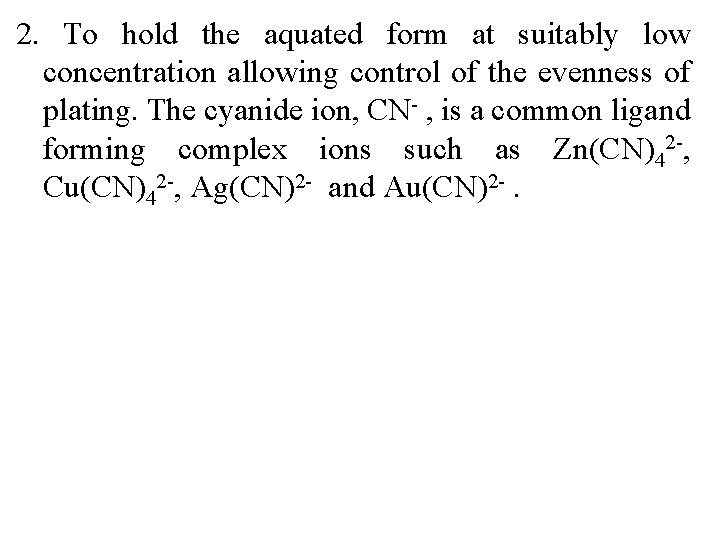
2. To hold the aquated form at suitably low concentration allowing control of the evenness of plating. The cyanide ion, CN- , is a common ligand forming complex ions such as Zn(CN)42 -, Cu(CN)42 -, Ag(CN)2 - and Au(CN)2 -.
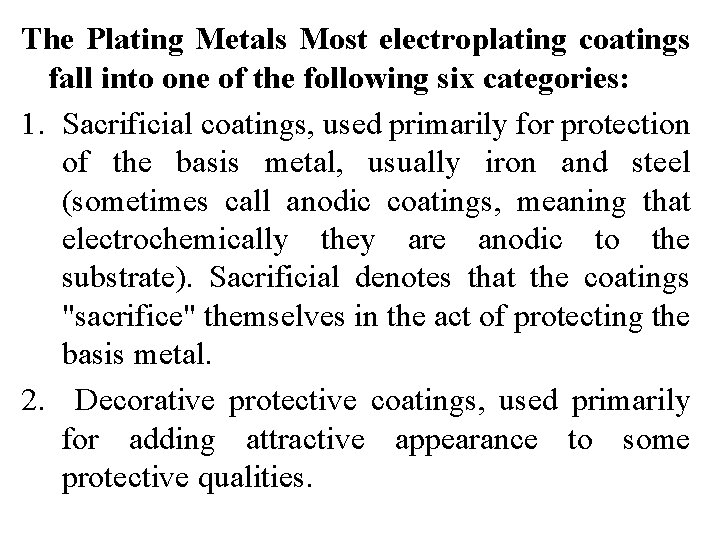
The Plating Metals Most electroplating coatings fall into one of the following six categories: 1. Sacrificial coatings, used primarily for protection of the basis metal, usually iron and steel (sometimes call anodic coatings, meaning that electrochemically they are anodic to the substrate). Sacrificial denotes that the coatings "sacrifice" themselves in the act of protecting the basis metal. 2. Decorative protective coatings, used primarily for adding attractive appearance to some protective qualities.
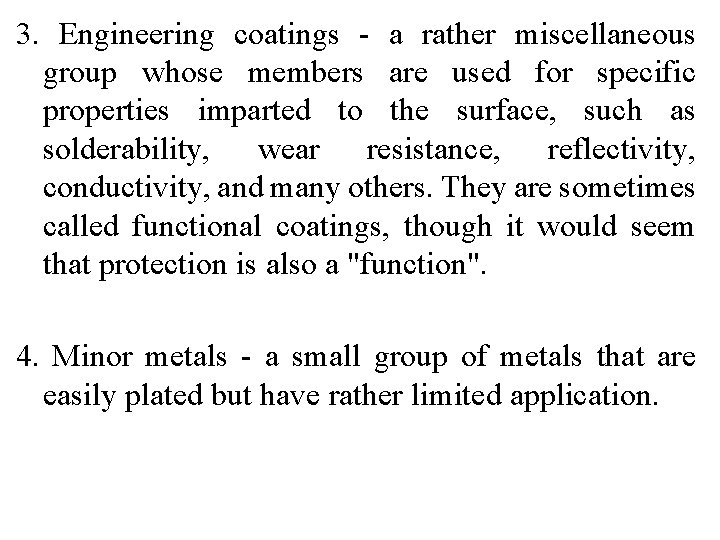
3. Engineering coatings - a rather miscellaneous group whose members are used for specific properties imparted to the surface, such as solderability, wear resistance, reflectivity, conductivity, and many others. They are sometimes called functional coatings, though it would seem that protection is also a "function". 4. Minor metals - a small group of metals that are easily plated but have rather limited application.
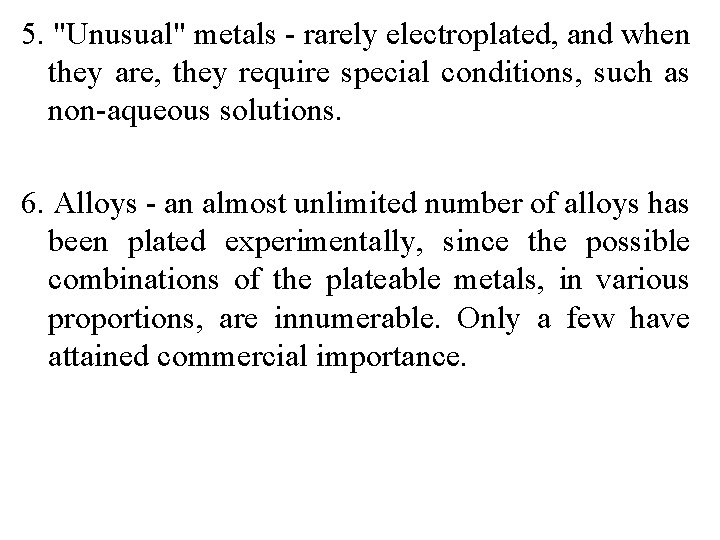
5. "Unusual" metals - rarely electroplated, and when they are, they require special conditions, such as non-aqueous solutions. 6. Alloys - an almost unlimited number of alloys has been plated experimentally, since the possible combinations of the plateable metals, in various proportions, are innumerable. Only a few have attained commercial importance.
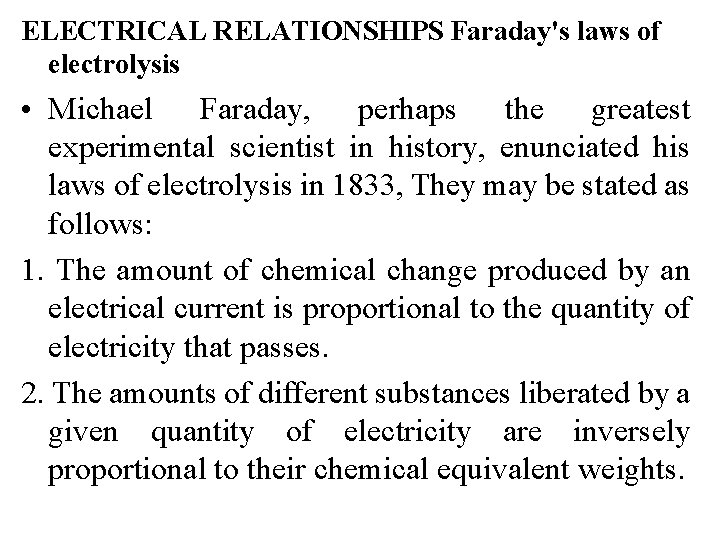
ELECTRICAL RELATIONSHIPS Faraday's laws of electrolysis • Michael Faraday, perhaps the greatest experimental scientist in history, enunciated his laws of electrolysis in 1833, They may be stated as follows: 1. The amount of chemical change produced by an electrical current is proportional to the quantity of electricity that passes. 2. The amounts of different substances liberated by a given quantity of electricity are inversely proportional to their chemical equivalent weights.
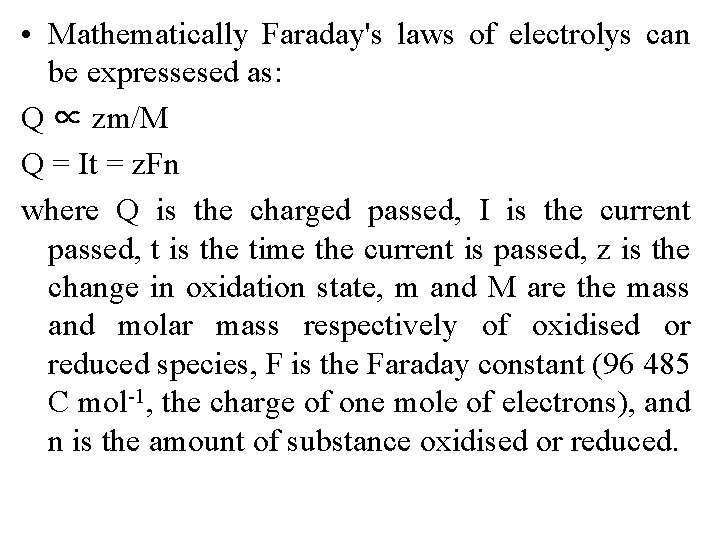
• Mathematically Faraday's laws of electrolys can be expressesed as: Q ∝ zm/M Q = It = z. Fn where Q is the charged passed, I is the current passed, t is the time the current is passed, z is the change in oxidation state, m and M are the mass and molar mass respectively of oxidised or reduced species, F is the Faraday constant (96 485 C mol-1, the charge of one mole of electrons), and n is the amount of substance oxidised or reduced.
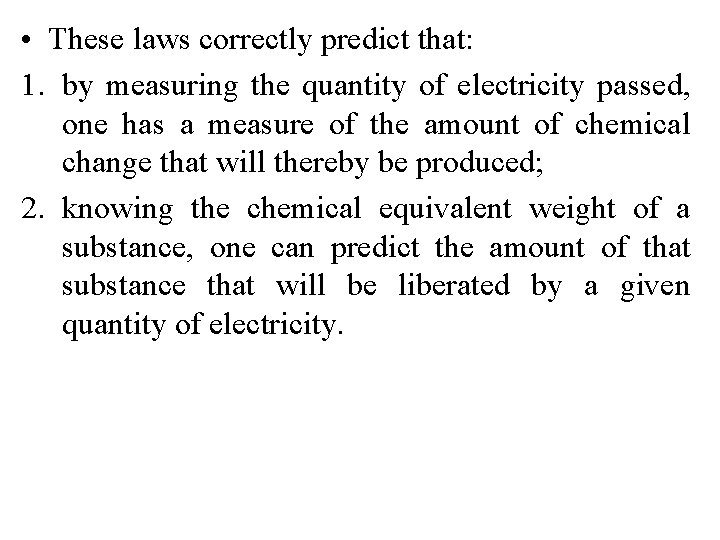
• These laws correctly predict that: 1. by measuring the quantity of electricity passed, one has a measure of the amount of chemical change that will thereby be produced; 2. knowing the chemical equivalent weight of a substance, one can predict the amount of that substance that will be liberated by a given quantity of electricity.
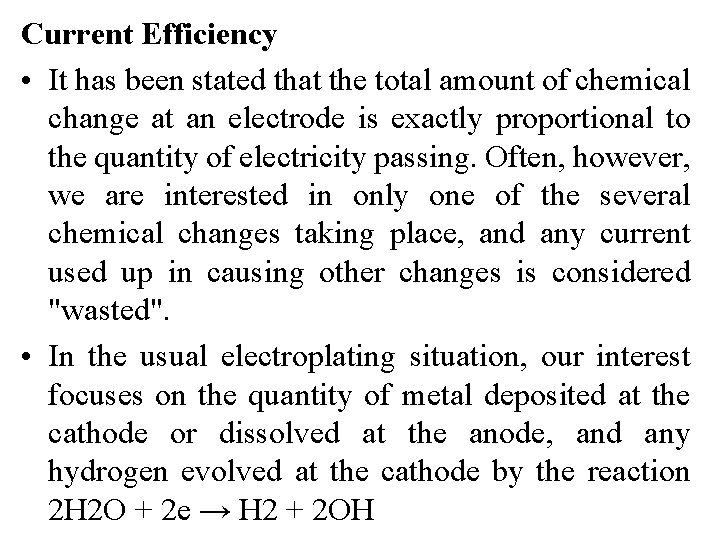
Current Efficiency • It has been stated that the total amount of chemical change at an electrode is exactly proportional to the quantity of electricity passing. Often, however, we are interested in only one of the several chemical changes taking place, and any current used up in causing other changes is considered "wasted". • In the usual electroplating situation, our interest focuses on the quantity of metal deposited at the cathode or dissolved at the anode, and any hydrogen evolved at the cathode by the reaction 2 H 2 O + 2 e → H 2 + 2 OH
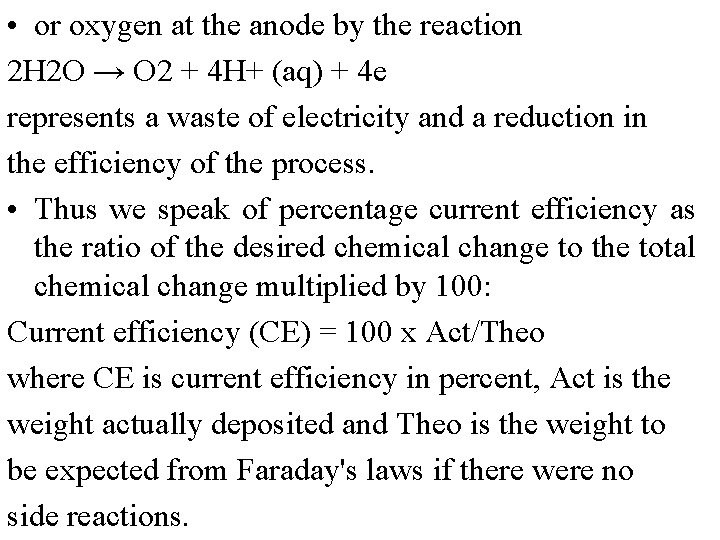
• or oxygen at the anode by the reaction 2 H 2 O → O 2 + 4 H+ (aq) + 4 e represents a waste of electricity and a reduction in the efficiency of the process. • Thus we speak of percentage current efficiency as the ratio of the desired chemical change to the total chemical change multiplied by 100: Current efficiency (CE) = 100 x Act/Theo where CE is current efficiency in percent, Act is the weight actually deposited and Theo is the weight to be expected from Faraday's laws if there were no side reactions.
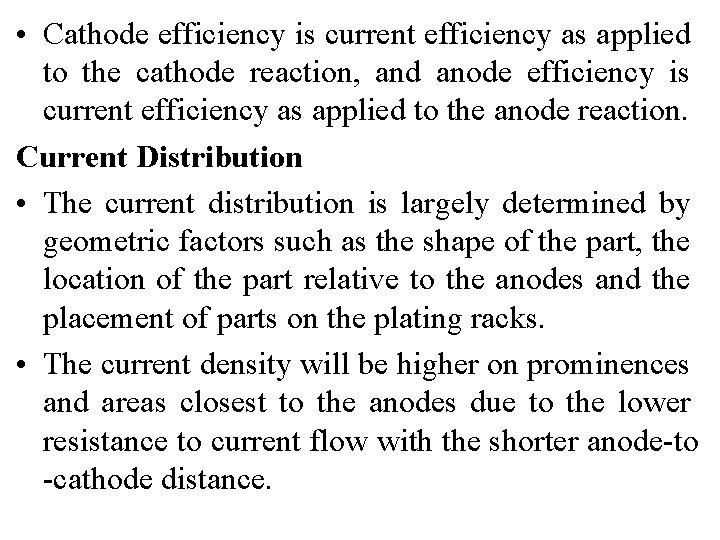
• Cathode efficiency is current efficiency as applied to the cathode reaction, and anode efficiency is current efficiency as applied to the anode reaction. Current Distribution • The current distribution is largely determined by geometric factors such as the shape of the part, the location of the part relative to the anodes and the placement of parts on the plating racks. • The current density will be higher on prominences and areas closest to the anodes due to the lower resistance to current flow with the shorter anode-to -cathode distance.
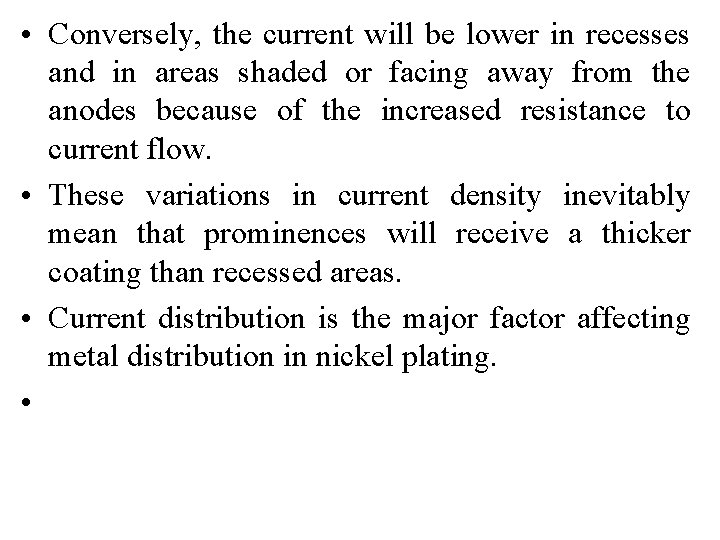
• Conversely, the current will be lower in recesses and in areas shaded or facing away from the anodes because of the increased resistance to current flow. • These variations in current density inevitably mean that prominences will receive a thicker coating than recessed areas. • Current distribution is the major factor affecting metal distribution in nickel plating. •
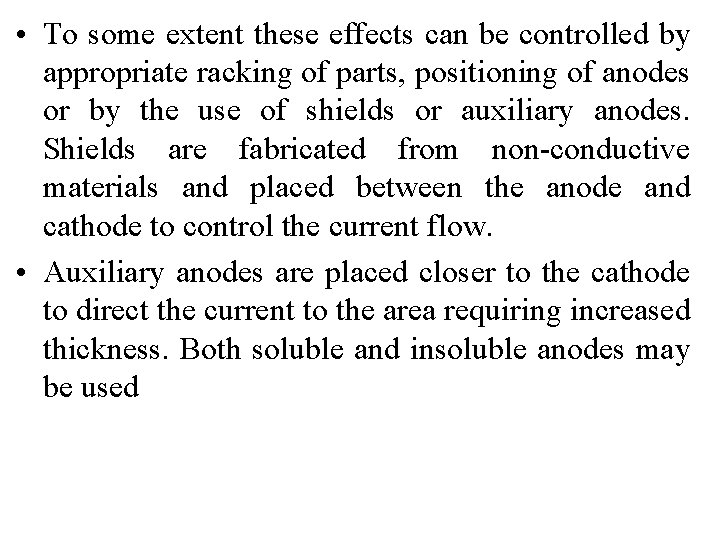
• To some extent these effects can be controlled by appropriate racking of parts, positioning of anodes or by the use of shields or auxiliary anodes. Shields are fabricated from non-conductive materials and placed between the anode and cathode to control the current flow. • Auxiliary anodes are placed closer to the cathode to direct the current to the area requiring increased thickness. Both soluble and insoluble anodes may be used
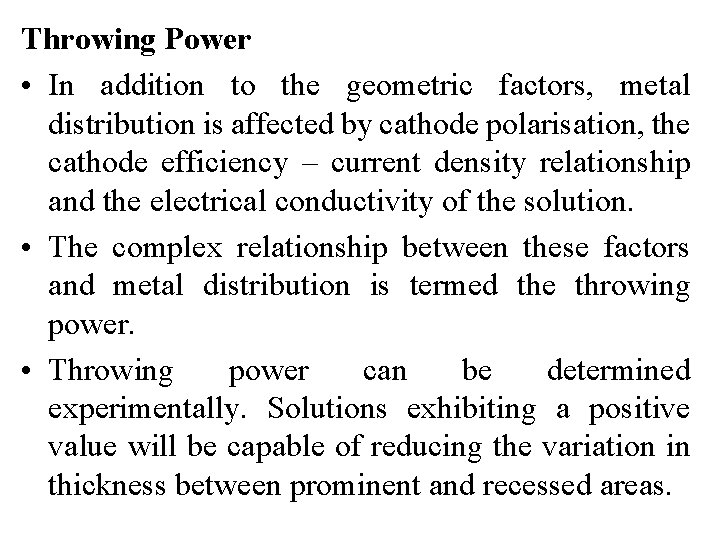
Throwing Power • In addition to the geometric factors, metal distribution is affected by cathode polarisation, the cathode efficiency – current density relationship and the electrical conductivity of the solution. • The complex relationship between these factors and metal distribution is termed the throwing power. • Throwing power can be determined experimentally. Solutions exhibiting a positive value will be capable of reducing the variation in thickness between prominent and recessed areas.
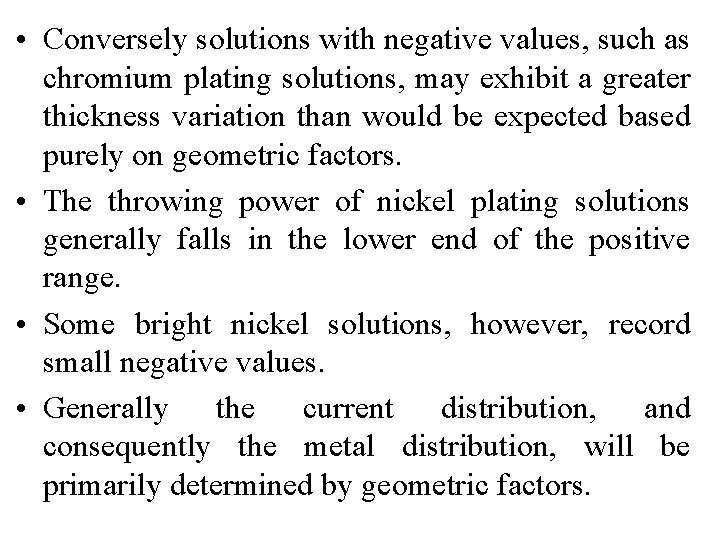
• Conversely solutions with negative values, such as chromium plating solutions, may exhibit a greater thickness variation than would be expected based purely on geometric factors. • The throwing power of nickel plating solutions generally falls in the lower end of the positive range. • Some bright nickel solutions, however, record small negative values. • Generally the current distribution, and consequently the metal distribution, will be primarily determined by geometric factors.
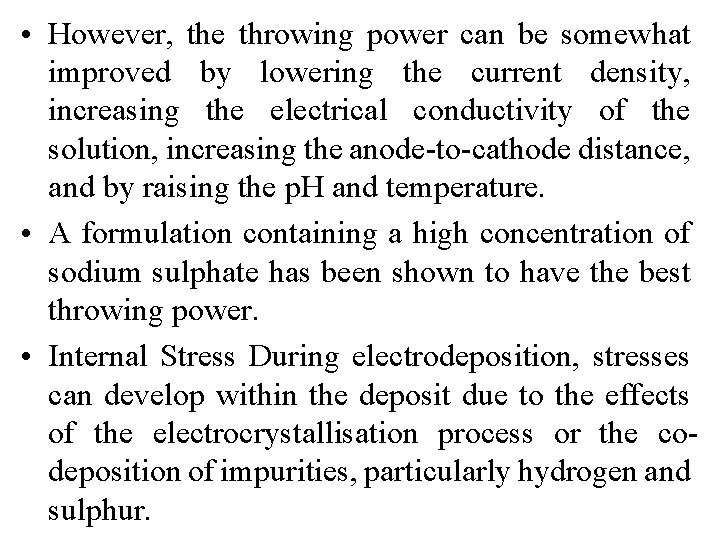
• However, the throwing power can be somewhat improved by lowering the current density, increasing the electrical conductivity of the solution, increasing the anode-to-cathode distance, and by raising the p. H and temperature. • A formulation containing a high concentration of sodium sulphate has been shown to have the best throwing power. • Internal Stress During electrodeposition, stresses can develop within the deposit due to the effects of the electrocrystallisation process or the codeposition of impurities, particularly hydrogen and sulphur.
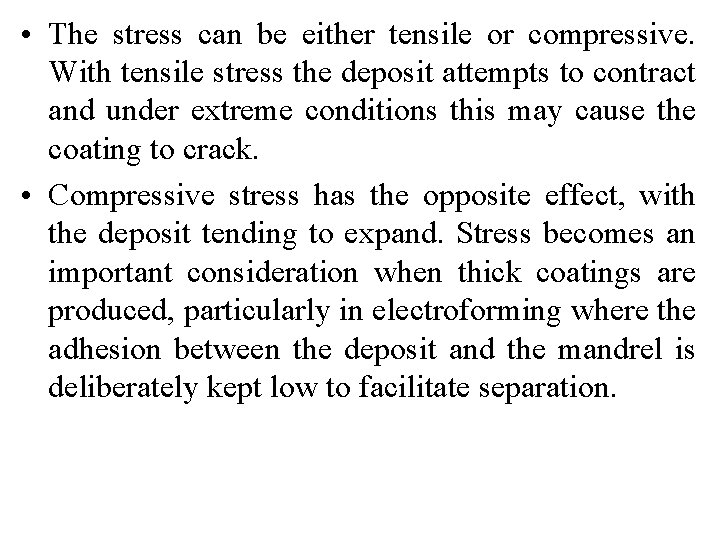
• The stress can be either tensile or compressive. With tensile stress the deposit attempts to contract and under extreme conditions this may cause the coating to crack. • Compressive stress has the opposite effect, with the deposit tending to expand. Stress becomes an important consideration when thick coatings are produced, particularly in electroforming where the adhesion between the deposit and the mandrel is deliberately kept low to facilitate separation.
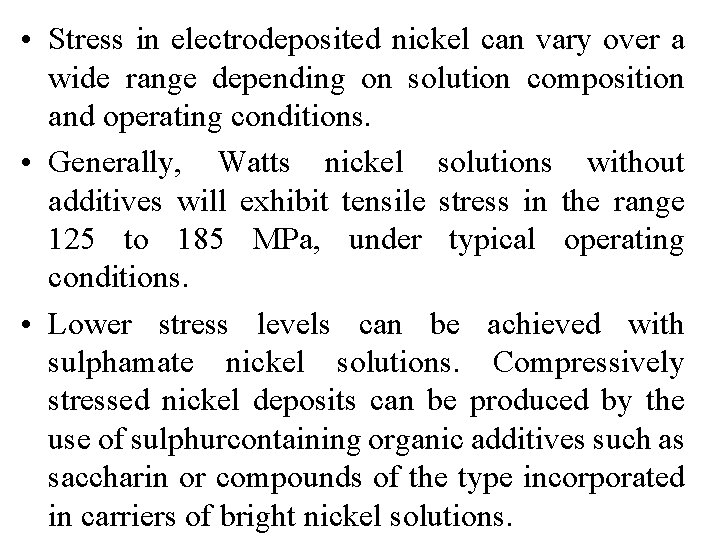
• Stress in electrodeposited nickel can vary over a wide range depending on solution composition and operating conditions. • Generally, Watts nickel solutions without additives will exhibit tensile stress in the range 125 to 185 MPa, under typical operating conditions. • Lower stress levels can be achieved with sulphamate nickel solutions. Compressively stressed nickel deposits can be produced by the use of sulphurcontaining organic additives such as saccharin or compounds of the type incorporated in carriers of bright nickel solutions.
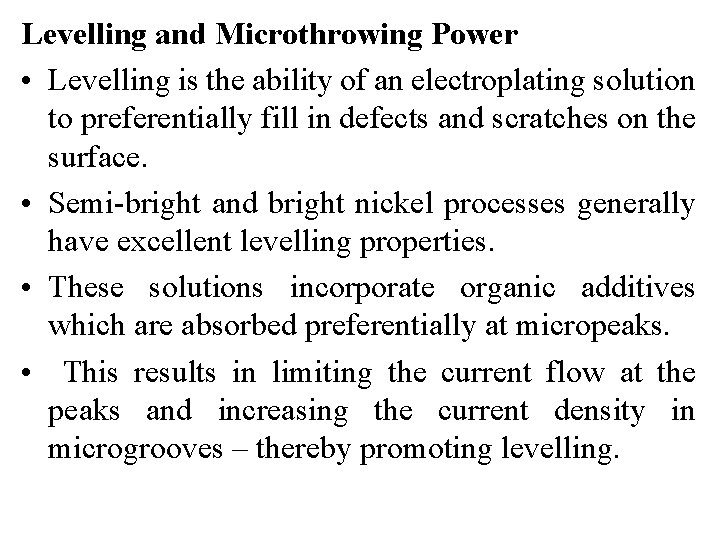
Levelling and Microthrowing Power • Levelling is the ability of an electroplating solution to preferentially fill in defects and scratches on the surface. • Semi-bright and bright nickel processes generally have excellent levelling properties. • These solutions incorporate organic additives which are absorbed preferentially at micropeaks. • This results in limiting the current flow at the peaks and increasing the current density in microgrooves – thereby promoting levelling.
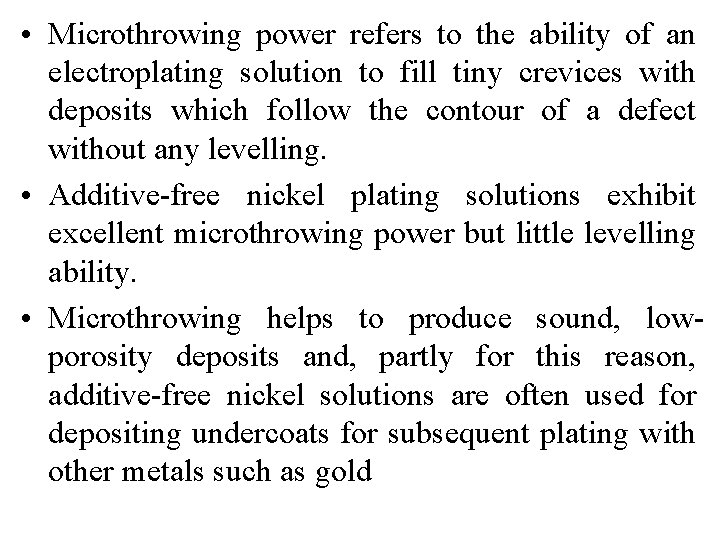
• Microthrowing power refers to the ability of an electroplating solution to fill tiny crevices with deposits which follow the contour of a defect without any levelling. • Additive-free nickel plating solutions exhibit excellent microthrowing power but little levelling ability. • Microthrowing helps to produce sound, lowporosity deposits and, partly for this reason, additive-free nickel solutions are often used for depositing undercoats for subsequent plating with other metals such as gold
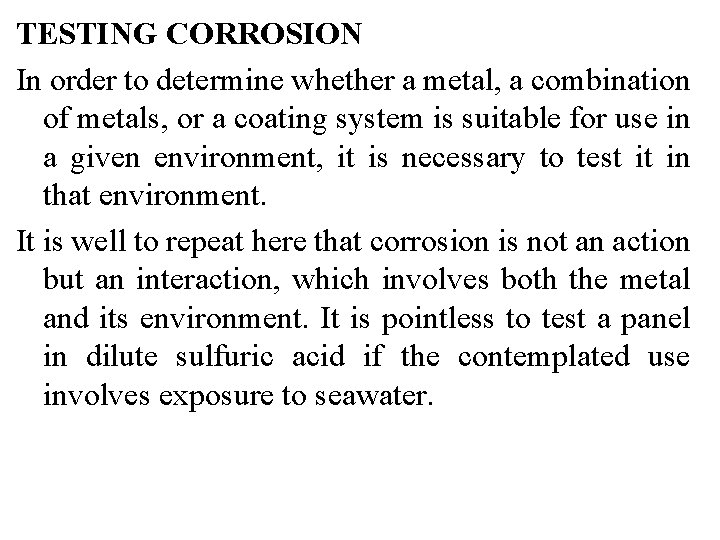
TESTING CORROSION In order to determine whether a metal, a combination of metals, or a coating system is suitable for use in a given environment, it is necessary to test it in that environment. It is well to repeat here that corrosion is not an action but an interaction, which involves both the metal and its environment. It is pointless to test a panel in dilute sulfuric acid if the contemplated use involves exposure to seawater.
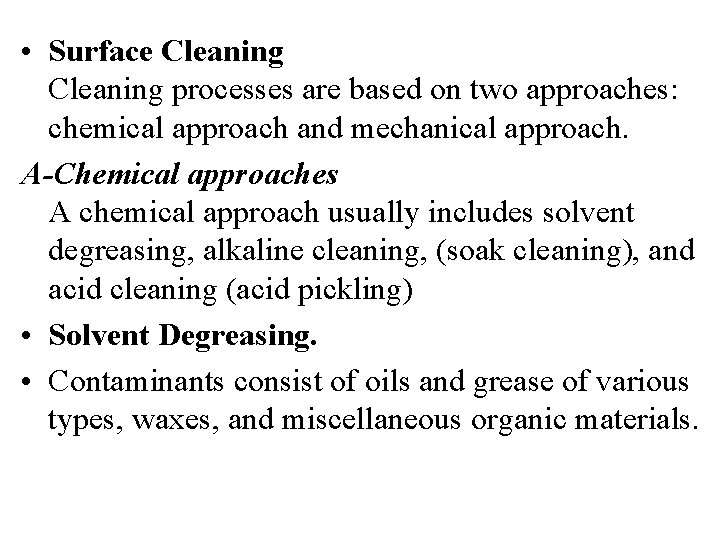
• Surface Cleaning processes are based on two approaches: chemical approach and mechanical approach. A-Chemical approaches A chemical approach usually includes solvent degreasing, alkaline cleaning, (soak cleaning), and acid cleaning (acid pickling) • Solvent Degreasing. • Contaminants consist of oils and grease of various types, waxes, and miscellaneous organic materials.
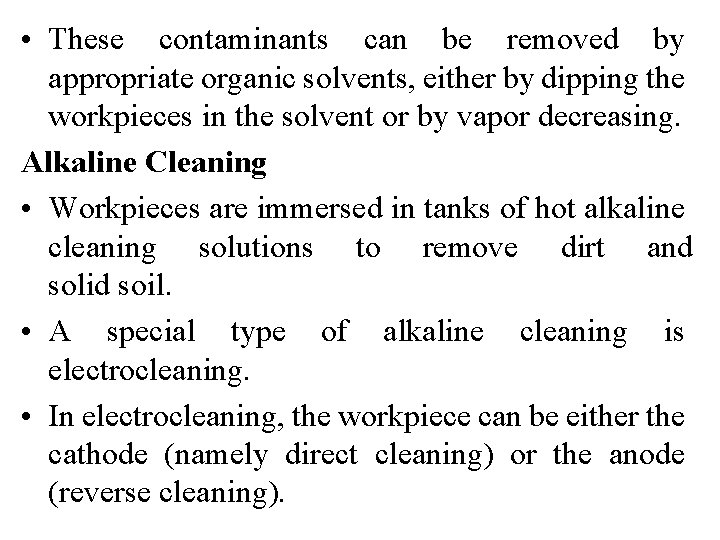
• These contaminants can be removed by appropriate organic solvents, either by dipping the workpieces in the solvent or by vapor decreasing. Alkaline Cleaning • Workpieces are immersed in tanks of hot alkaline cleaning solutions to remove dirt and solid soil. • A special type of alkaline cleaning is electrocleaning. • In electrocleaning, the workpiece can be either the cathode (namely direct cleaning) or the anode (reverse cleaning).
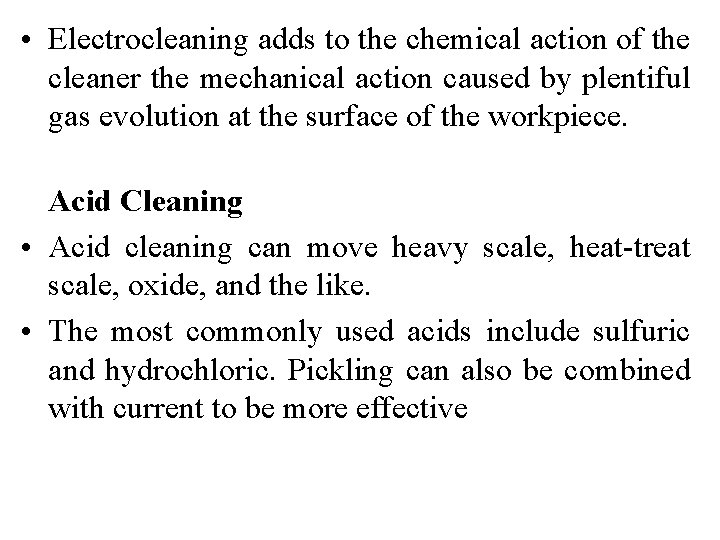
• Electrocleaning adds to the chemical action of the cleaner the mechanical action caused by plentiful gas evolution at the surface of the workpiece. Acid Cleaning • Acid cleaning can move heavy scale, heat-treat scale, oxide, and the like. • The most commonly used acids include sulfuric and hydrochloric. Pickling can also be combined with current to be more effective
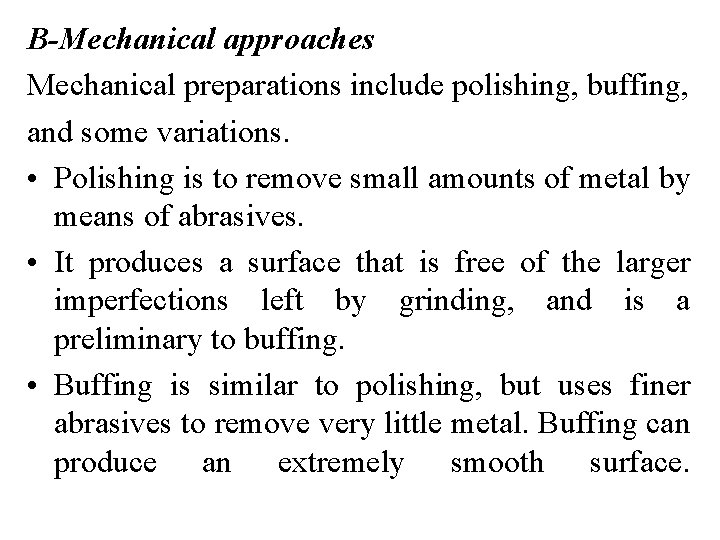
B-Mechanical approaches Mechanical preparations include polishing, buffing, and some variations. • Polishing is to remove small amounts of metal by means of abrasives. • It produces a surface that is free of the larger imperfections left by grinding, and is a preliminary to buffing. • Buffing is similar to polishing, but uses finer abrasives to remove very little metal. Buffing can produce an extremely smooth surface.
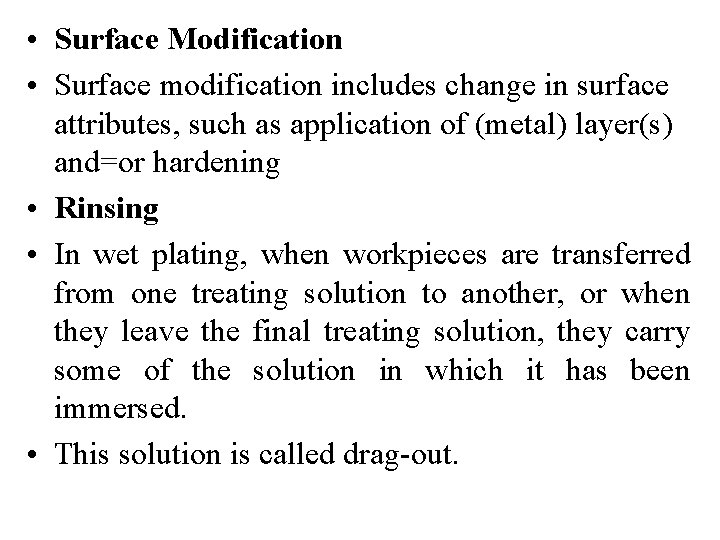
• Surface Modification • Surface modification includes change in surface attributes, such as application of (metal) layer(s) and=or hardening • Rinsing • In wet plating, when workpieces are transferred from one treating solution to another, or when they leave the final treating solution, they carry some of the solution in which it has been immersed. • This solution is called drag-out.
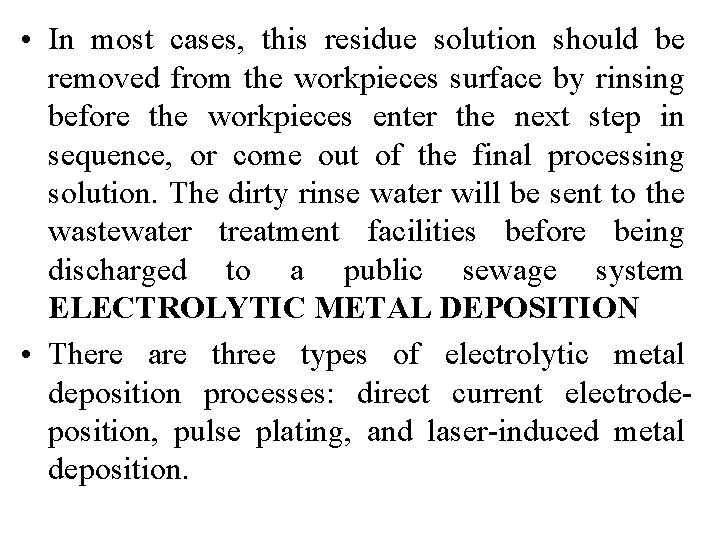
• In most cases, this residue solution should be removed from the workpieces surface by rinsing before the workpieces enter the next step in sequence, or come out of the final processing solution. The dirty rinse water will be sent to the wastewater treatment facilities before being discharged to a public sewage system ELECTROLYTIC METAL DEPOSITION • There are three types of electrolytic metal deposition processes: direct current electrodeposition, pulse plating, and laser-induced metal deposition.
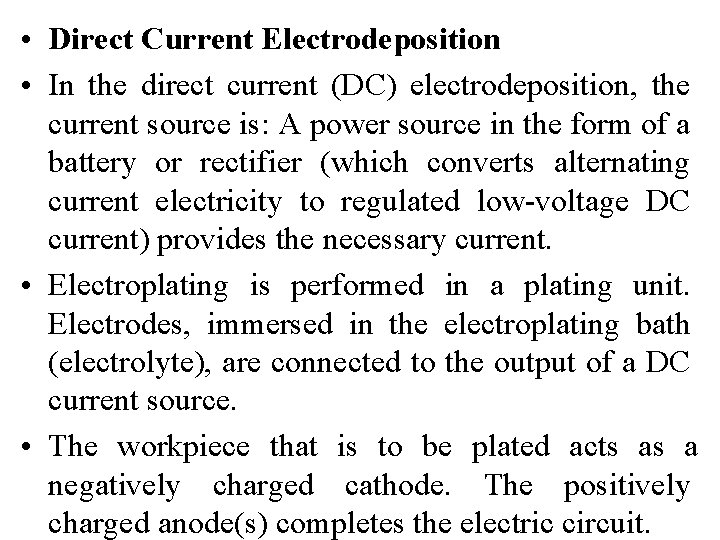
• Direct Current Electrodeposition • In the direct current (DC) electrodeposition, the current source is: A power source in the form of a battery or rectifier (which converts alternating current electricity to regulated low-voltage DC current) provides the necessary current. • Electroplating is performed in a plating unit. Electrodes, immersed in the electroplating bath (electrolyte), are connected to the output of a DC current source. • The workpiece that is to be plated acts as a negatively charged cathode. The positively charged anode(s) completes the electric circuit.
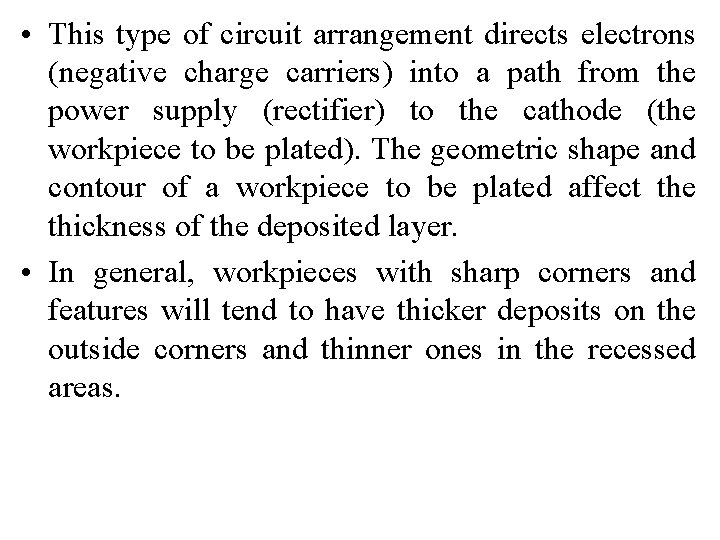
• This type of circuit arrangement directs electrons (negative charge carriers) into a path from the power supply (rectifier) to the cathode (the workpiece to be plated). The geometric shape and contour of a workpiece to be plated affect the thickness of the deposited layer. • In general, workpieces with sharp corners and features will tend to have thicker deposits on the outside corners and thinner ones in the recessed areas.
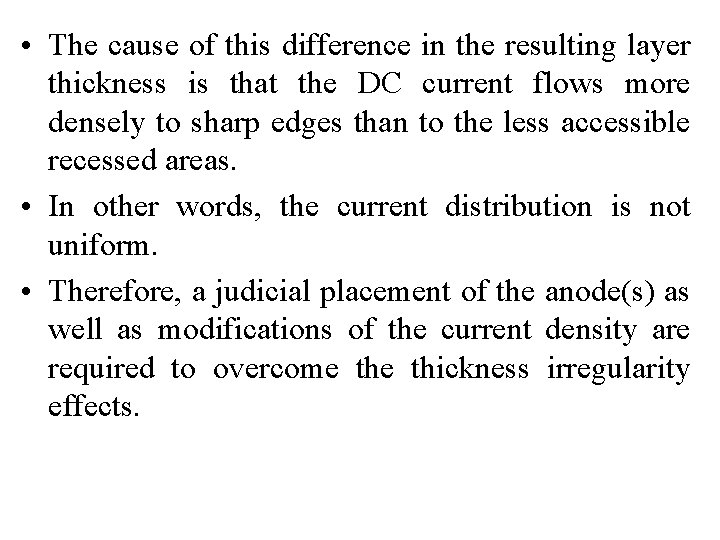
• The cause of this difference in the resulting layer thickness is that the DC current flows more densely to sharp edges than to the less accessible recessed areas. • In other words, the current distribution is not uniform. • Therefore, a judicial placement of the anode(s) as well as modifications of the current density are required to overcome thickness irregularity effects.
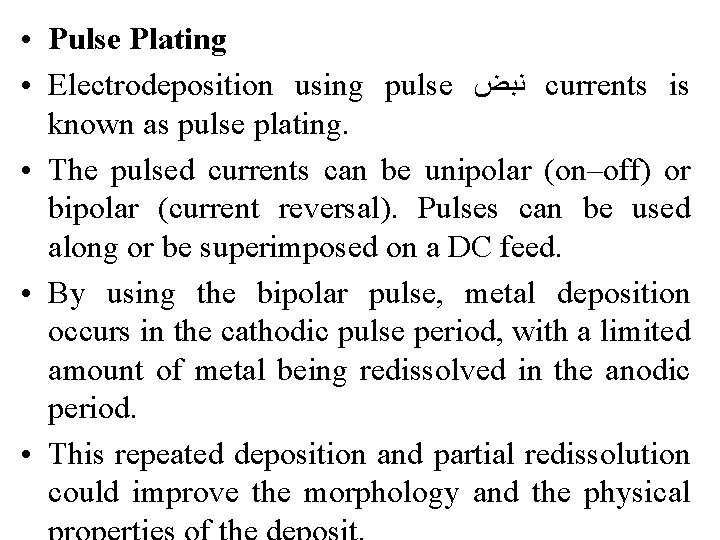
• Pulse Plating • Electrodeposition using pulse ﻧﺒﺾ currents is known as pulse plating. • The pulsed currents can be unipolar (on–off) or bipolar (current reversal). Pulses can be used along or be superimposed on a DC feed. • By using the bipolar pulse, metal deposition occurs in the cathodic pulse period, with a limited amount of metal being redissolved in the anodic period. • This repeated deposition and partial redissolution could improve the morphology and the physical
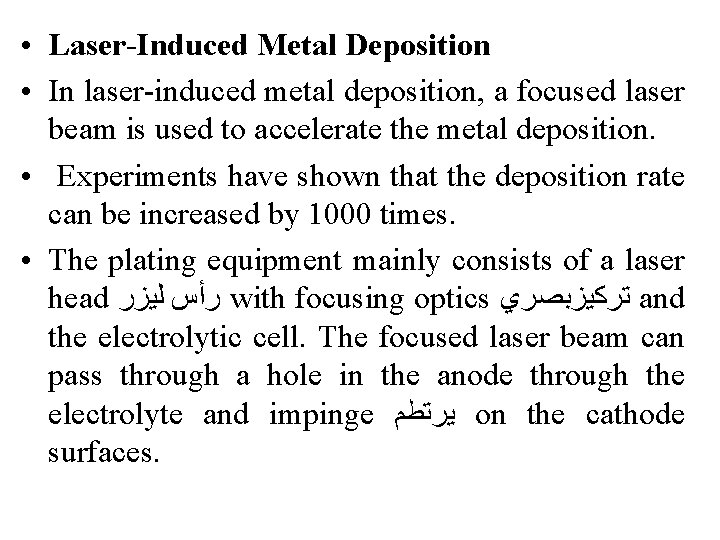
• Laser-Induced Metal Deposition • In laser-induced metal deposition, a focused laser beam is used to accelerate the metal deposition. • Experiments have shown that the deposition rate can be increased by 1000 times. • The plating equipment mainly consists of a laser head ﺭﺃﺲ ﻟﻴﺰﺭ with focusing optics ﺗﺮﻛﻴﺰﺑﺼﺮﻱ and the electrolytic cell. The focused laser beam can pass through a hole in the anode through the electrolyte and impinge ﻳﺮﺗﻄﻢ on the cathode surfaces.
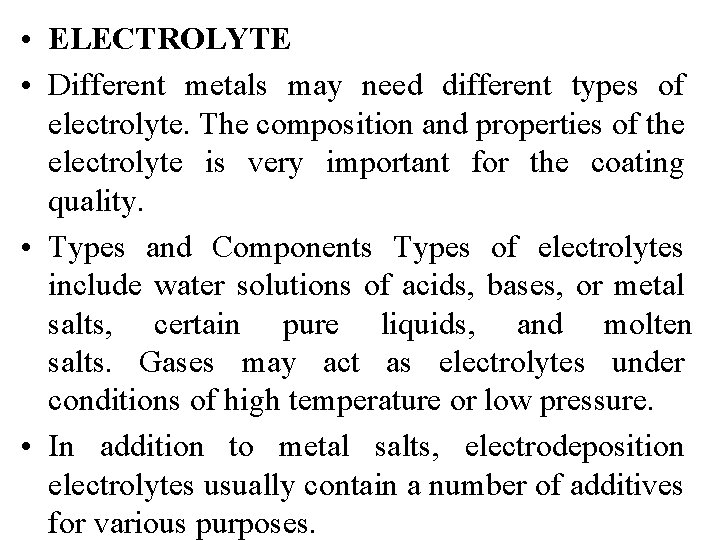
• ELECTROLYTE • Different metals may need different types of electrolyte. The composition and properties of the electrolyte is very important for the coating quality. • Types and Components Types of electrolytes include water solutions of acids, bases, or metal salts, certain pure liquids, and molten salts. Gases may act as electrolytes under conditions of high temperature or low pressure. • In addition to metal salts, electrodeposition electrolytes usually contain a number of additives for various purposes.
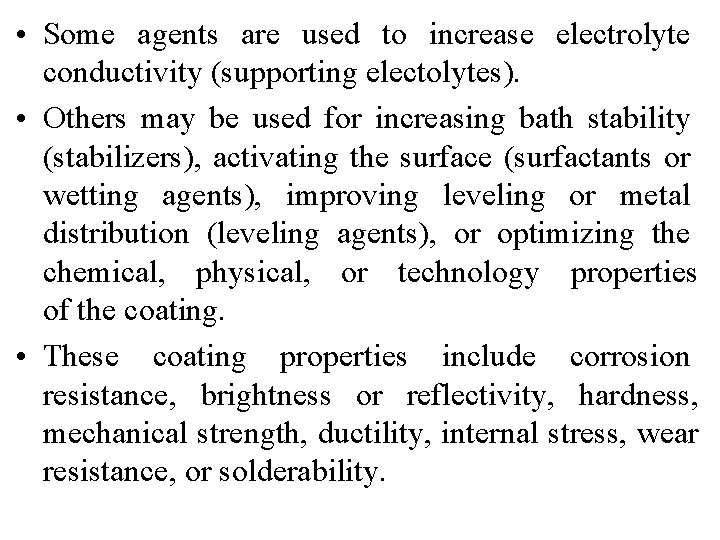
• Some agents are used to increase electrolyte conductivity (supporting electolytes). • Others may be used for increasing bath stability (stabilizers), activating the surface (surfactants or wetting agents), improving leveling or metal distribution (leveling agents), or optimizing the chemical, physical, or technology properties of the coating. • These coating properties include corrosion resistance, brightness or reflectivity, hardness, mechanical strength, ductility, internal stress, wear resistance, or solderability.
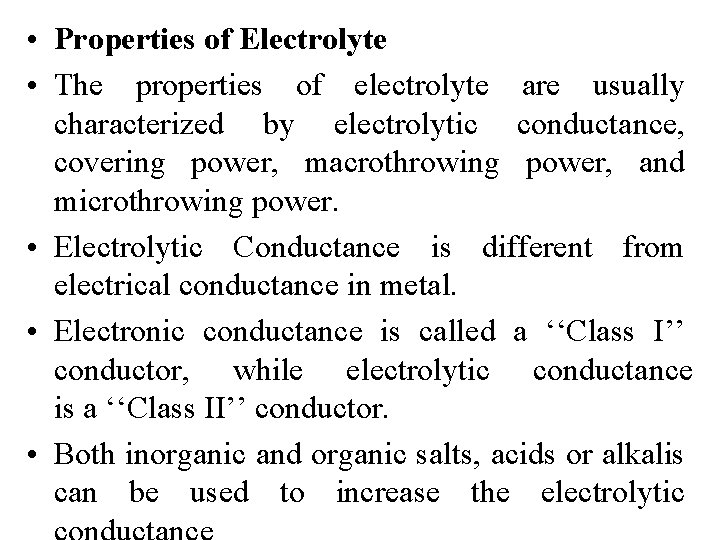
• Properties of Electrolyte • The properties of electrolyte are usually characterized by electrolytic conductance, covering power, macrothrowing power, and microthrowing power. • Electrolytic Conductance is different from electrical conductance in metal. • Electronic conductance is called a ‘‘Class I’’ conductor, while electrolytic conductance is a ‘‘Class II’’ conductor. • Both inorganic and organic salts, acids or alkalis can be used to increase the electrolytic
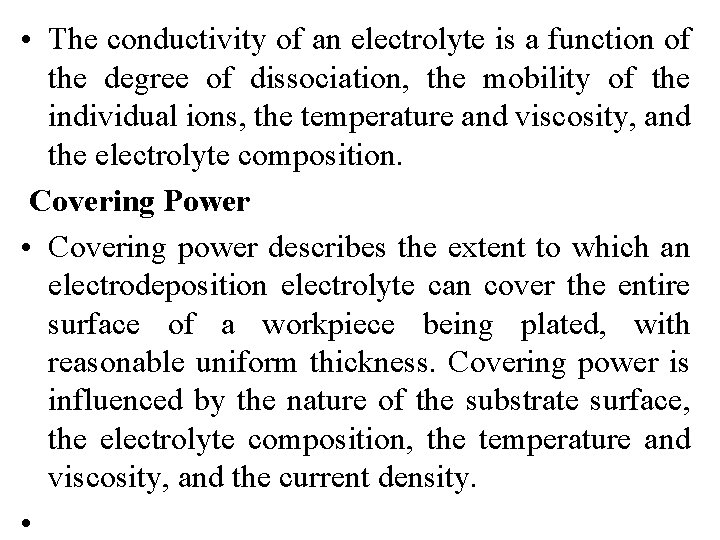
• The conductivity of an electrolyte is a function of the degree of dissociation, the mobility of the individual ions, the temperature and viscosity, and the electrolyte composition. Covering Power • Covering power describes the extent to which an electrodeposition electrolyte can cover the entire surface of a workpiece being plated, with reasonable uniform thickness. Covering power is influenced by the nature of the substrate surface, the electrolyte composition, the temperature and viscosity, and the current density. •
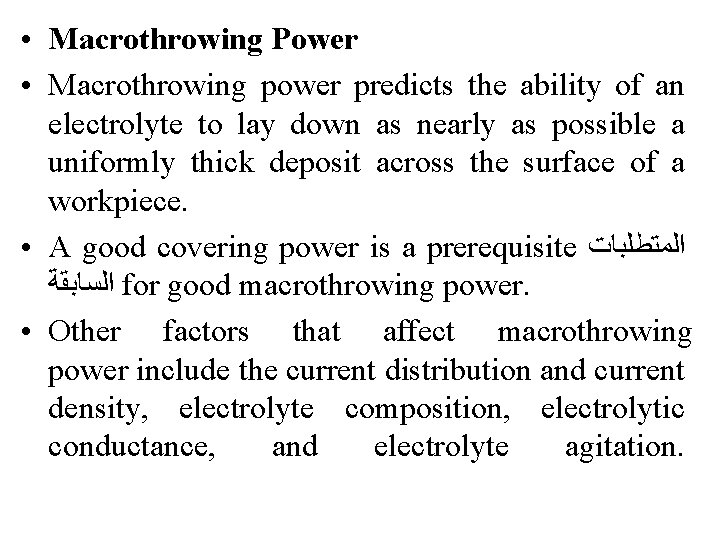
• Macrothrowing Power • Macrothrowing power predicts the ability of an electrolyte to lay down as nearly as possible a uniformly thick deposit across the surface of a workpiece. • A good covering power is a prerequisite ﺍﻟﻤﺘﻄﻠﺒﺎﺕ ﺍﻟﺴﺎﺑﻘﺔ for good macrothrowing power. • Other factors that affect macrothrowing power include the current distribution and current density, electrolyte composition, electrolytic conductance, and electrolyte agitation.
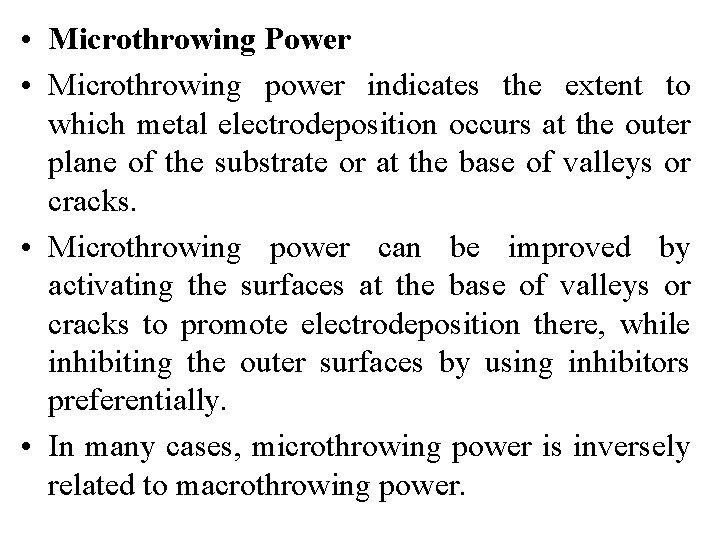
• Microthrowing Power • Microthrowing power indicates the extent to which metal electrodeposition occurs at the outer plane of the substrate or at the base of valleys or cracks. • Microthrowing power can be improved by activating the surfaces at the base of valleys or cracks to promote electrodeposition there, while inhibiting the outer surfaces by using inhibitors preferentially. • In many cases, microthrowing power is inversely related to macrothrowing power.
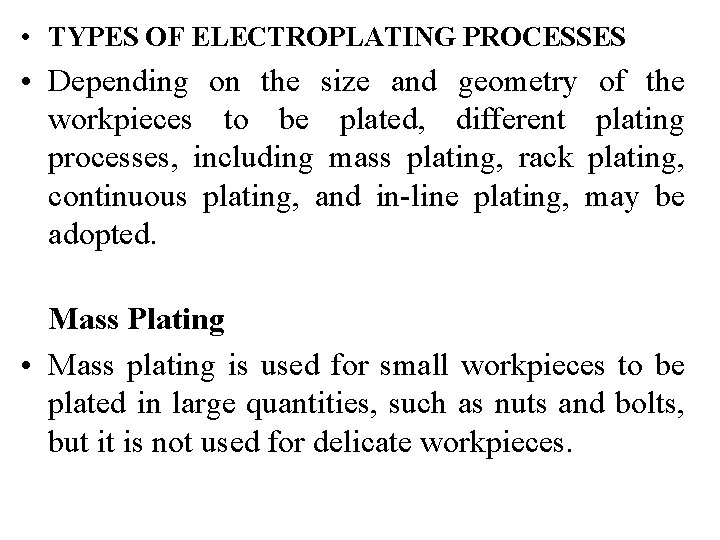
• TYPES OF ELECTROPLATING PROCESSES • Depending on the size and geometry of the workpieces to be plated, different plating processes, including mass plating, rack plating, continuous plating, and in-line plating, may be adopted. Mass Plating • Mass plating is used for small workpieces to be plated in large quantities, such as nuts and bolts, but it is not used for delicate workpieces.
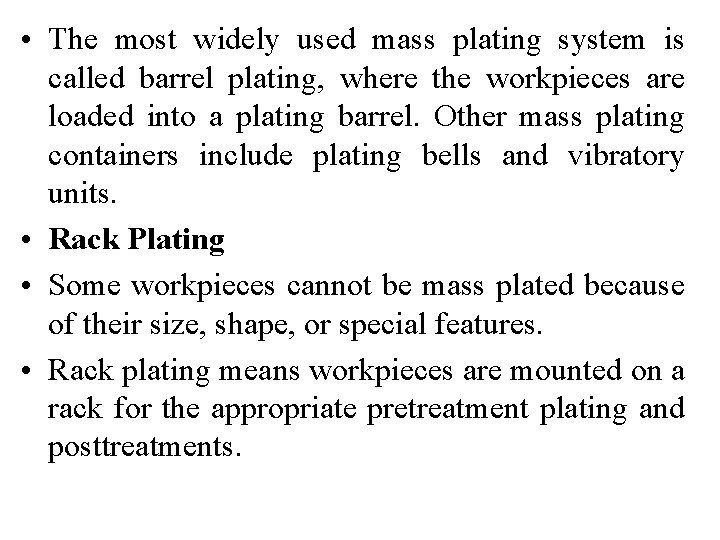
• The most widely used mass plating system is called barrel plating, where the workpieces are loaded into a plating barrel. Other mass plating containers include plating bells and vibratory units. • Rack Plating • Some workpieces cannot be mass plated because of their size, shape, or special features. • Rack plating means workpieces are mounted on a rack for the appropriate pretreatment plating and posttreatments.
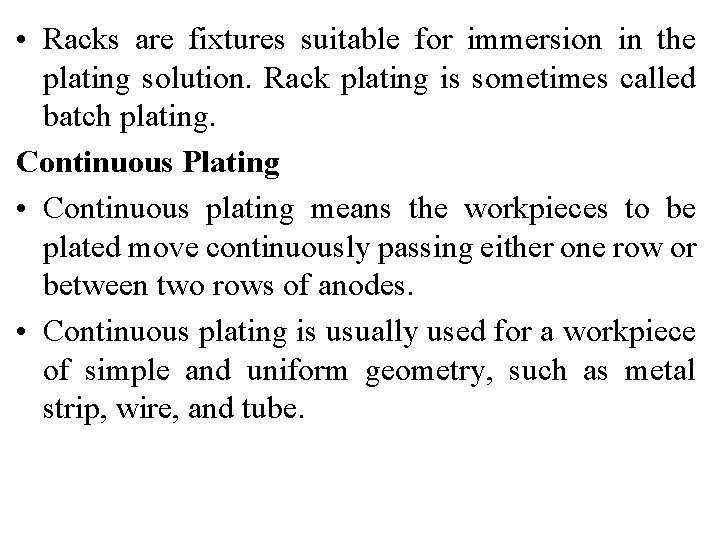
• Racks are fixtures suitable for immersion in the plating solution. Rack plating is sometimes called batch plating. Continuous Plating • Continuous plating means the workpieces to be plated move continuously passing either one row or between two rows of anodes. • Continuous plating is usually used for a workpiece of simple and uniform geometry, such as metal strip, wire, and tube.
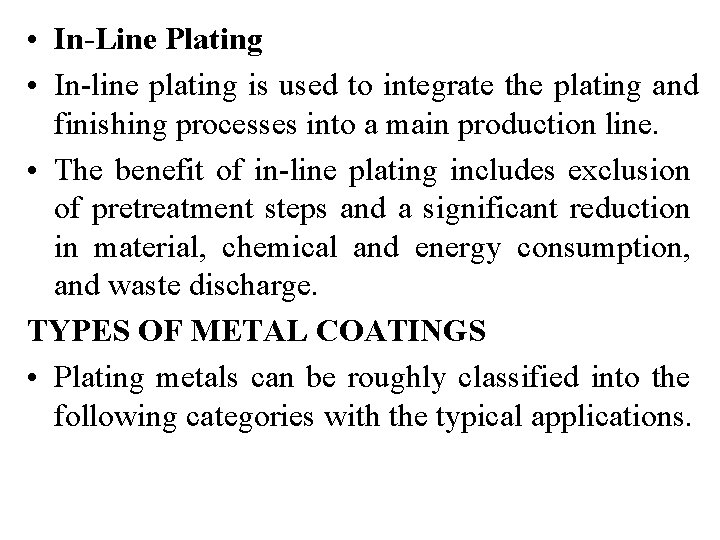
• In-Line Plating • In-line plating is used to integrate the plating and finishing processes into a main production line. • The benefit of in-line plating includes exclusion of pretreatment steps and a significant reduction in material, chemical and energy consumption, and waste discharge. TYPES OF METAL COATINGS • Plating metals can be roughly classified into the following categories with the typical applications.
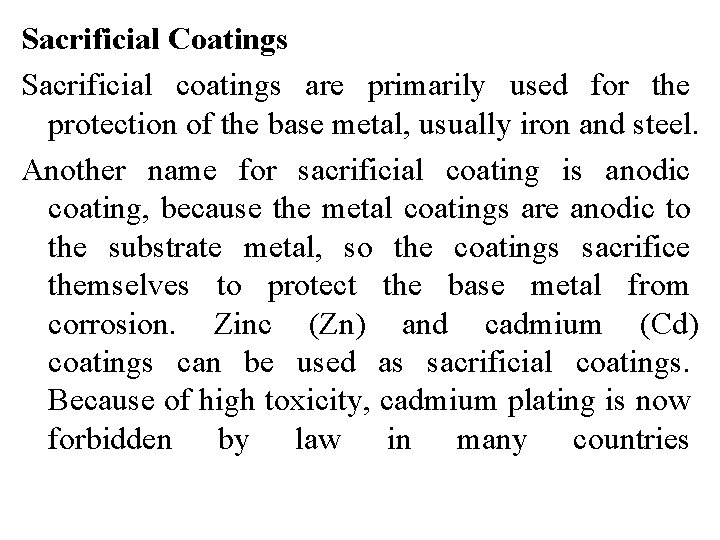
Sacrificial Coatings Sacrificial coatings are primarily used for the protection of the base metal, usually iron and steel. Another name for sacrificial coating is anodic coating, because the metal coatings are anodic to the substrate metal, so the coatings sacrifice themselves to protect the base metal from corrosion. Zinc (Zn) and cadmium (Cd) coatings can be used as sacrificial coatings. Because of high toxicity, cadmium plating is now forbidden by law in many countries
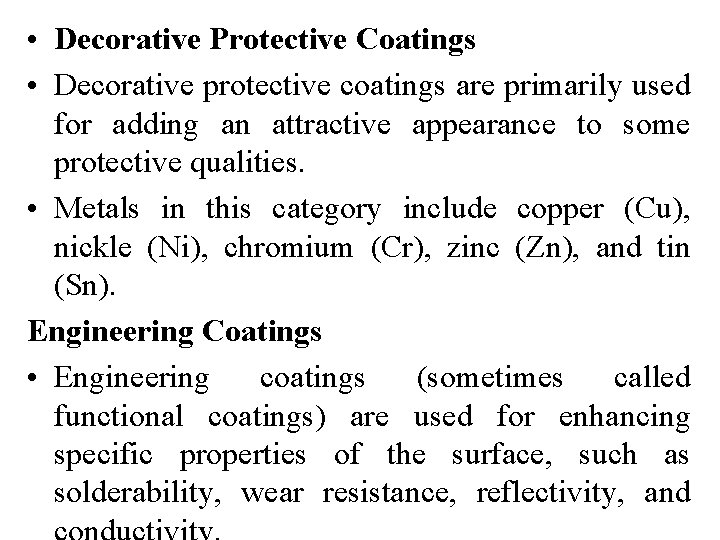
• Decorative Protective Coatings • Decorative protective coatings are primarily used for adding an attractive appearance to some protective qualities. • Metals in this category include copper (Cu), nickle (Ni), chromium (Cr), zinc (Zn), and tin (Sn). Engineering Coatings • Engineering coatings (sometimes called functional coatings) are used for enhancing specific properties of the surface, such as solderability, wear resistance, reflectivity, and
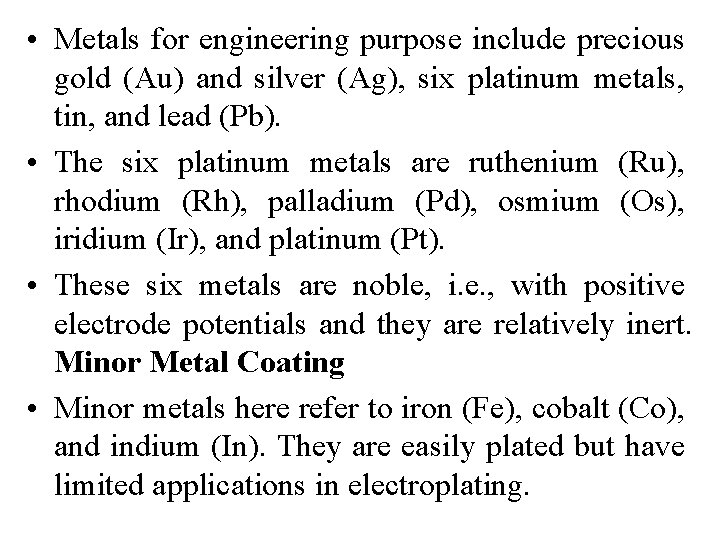
• Metals for engineering purpose include precious gold (Au) and silver (Ag), six platinum metals, tin, and lead (Pb). • The six platinum metals are ruthenium (Ru), rhodium (Rh), palladium (Pd), osmium (Os), iridium (Ir), and platinum (Pt). • These six metals are noble, i. e. , with positive electrode potentials and they are relatively inert. Minor Metal Coating • Minor metals here refer to iron (Fe), cobalt (Co), and indium (In). They are easily plated but have limited applications in electroplating.
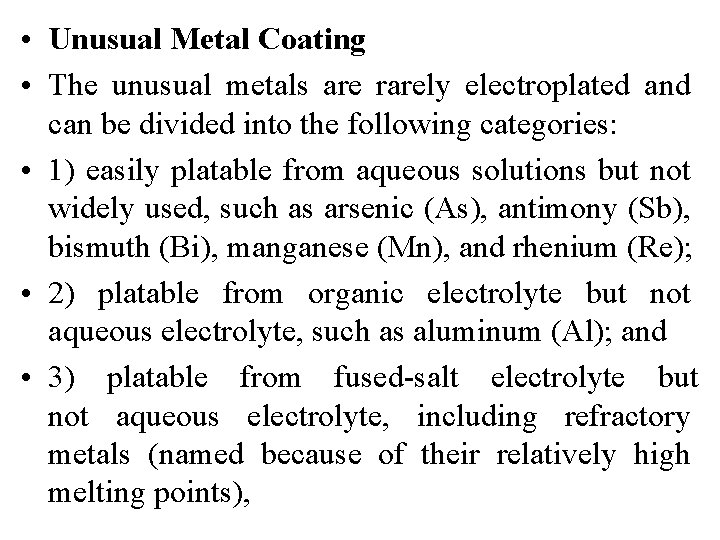
• Unusual Metal Coating • The unusual metals are rarely electroplated and can be divided into the following categories: • 1) easily platable from aqueous solutions but not widely used, such as arsenic (As), antimony (Sb), bismuth (Bi), manganese (Mn), and rhenium (Re); • 2) platable from organic electrolyte but not aqueous electrolyte, such as aluminum (Al); and • 3) platable from fused-salt electrolyte but not aqueous electrolyte, including refractory metals (named because of their relatively high melting points),
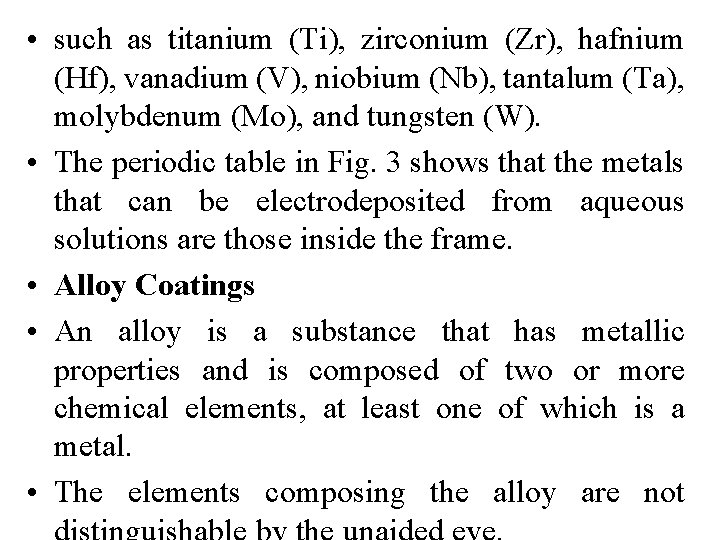
• such as titanium (Ti), zirconium (Zr), hafnium (Hf), vanadium (V), niobium (Nb), tantalum (Ta), molybdenum (Mo), and tungsten (W). • The periodic table in Fig. 3 shows that the metals that can be electrodeposited from aqueous solutions are those inside the frame. • Alloy Coatings • An alloy is a substance that has metallic properties and is composed of two or more chemical elements, at least one of which is a metal. • The elements composing the alloy are not
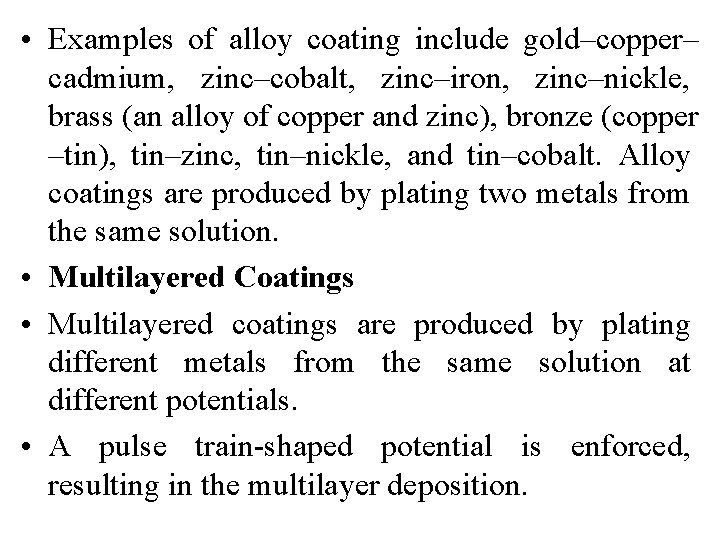
• Examples of alloy coating include gold–copper– cadmium, zinc–cobalt, zinc–iron, zinc–nickle, brass (an alloy of copper and zinc), bronze (copper –tin), tin–zinc, tin–nickle, and tin–cobalt. Alloy coatings are produced by plating two metals from the same solution. • Multilayered Coatings • Multilayered coatings are produced by plating different metals from the same solution at different potentials. • A pulse train-shaped potential is enforced, resulting in the multilayer deposition.
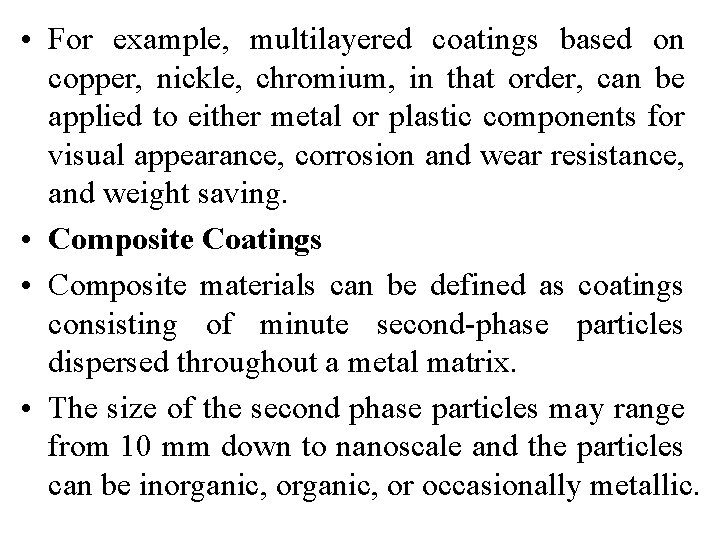
• For example, multilayered coatings based on copper, nickle, chromium, in that order, can be applied to either metal or plastic components for visual appearance, corrosion and wear resistance, and weight saving. • Composite Coatings • Composite materials can be defined as coatings consisting of minute second-phase particles dispersed throughout a metal matrix. • The size of the second phase particles may range from 10 mm down to nanoscale and the particles can be inorganic, or occasionally metallic.
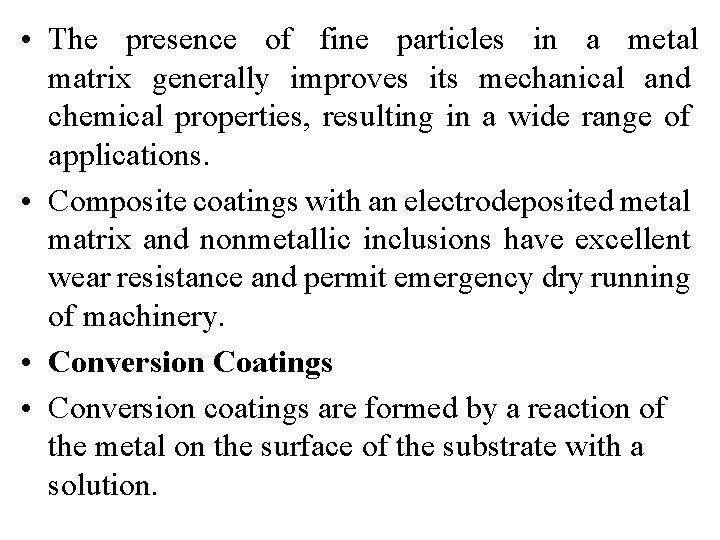
• The presence of fine particles in a metal matrix generally improves its mechanical and chemical properties, resulting in a wide range of applications. • Composite coatings with an electrodeposited metal matrix and nonmetallic inclusions have excellent wear resistance and permit emergency dry running of machinery. • Conversion Coatings • Conversion coatings are formed by a reaction of the metal on the surface of the substrate with a solution.
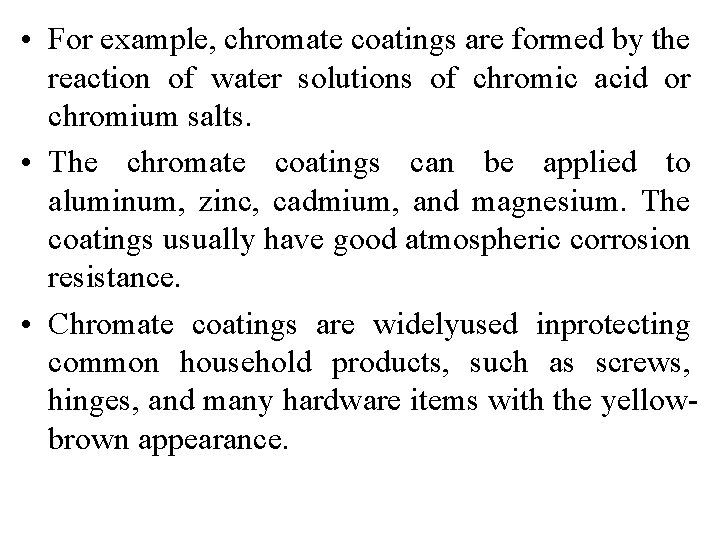
• For example, chromate coatings are formed by the reaction of water solutions of chromic acid or chromium salts. • The chromate coatings can be applied to aluminum, zinc, cadmium, and magnesium. The coatings usually have good atmospheric corrosion resistance. • Chromate coatings are widelyused inprotecting common household products, such as screws, hinges, and many hardware items with the yellowbrown appearance.
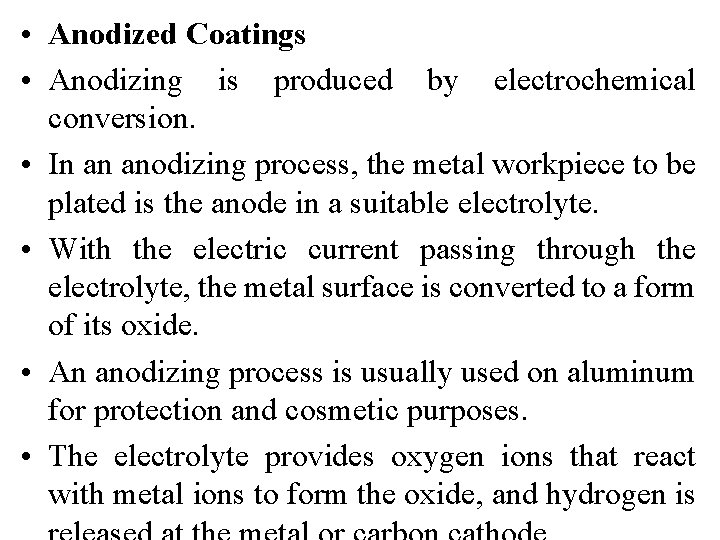
• Anodized Coatings • Anodizing is produced by electrochemical conversion. • In an anodizing process, the metal workpiece to be plated is the anode in a suitable electrolyte. • With the electric current passing through the electrolyte, the metal surface is converted to a form of its oxide. • An anodizing process is usually used on aluminum for protection and cosmetic purposes. • The electrolyte provides oxygen ions that react with metal ions to form the oxide, and hydrogen is
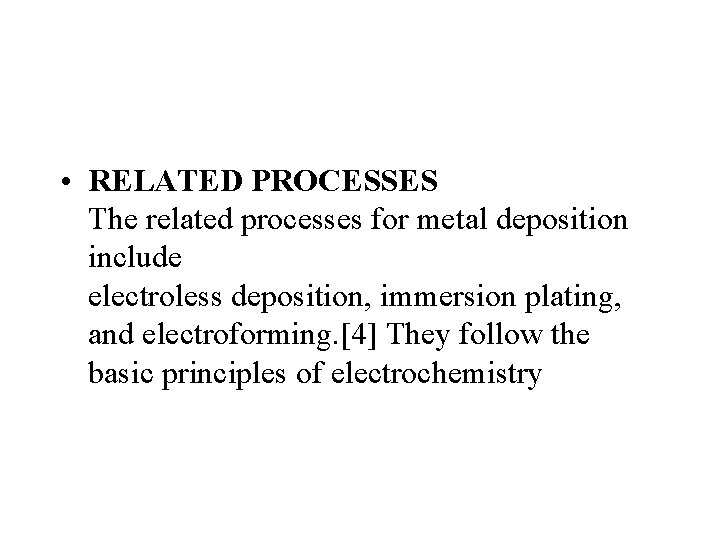
• RELATED PROCESSES The related processes for metal deposition include electroless deposition, immersion plating, and electroforming. [4] They follow the basic principles of electrochemistry
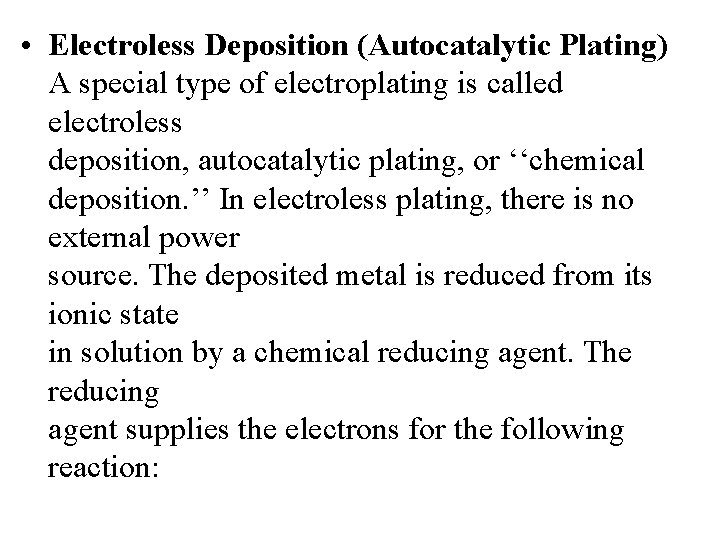
• Electroless Deposition (Autocatalytic Plating) A special type of electroplating is called electroless deposition, autocatalytic plating, or ‘‘chemical deposition. ’’ In electroless plating, there is no external power source. The deposited metal is reduced from its ionic state in solution by a chemical reducing agent. The reducing agent supplies the electrons for the following reaction:
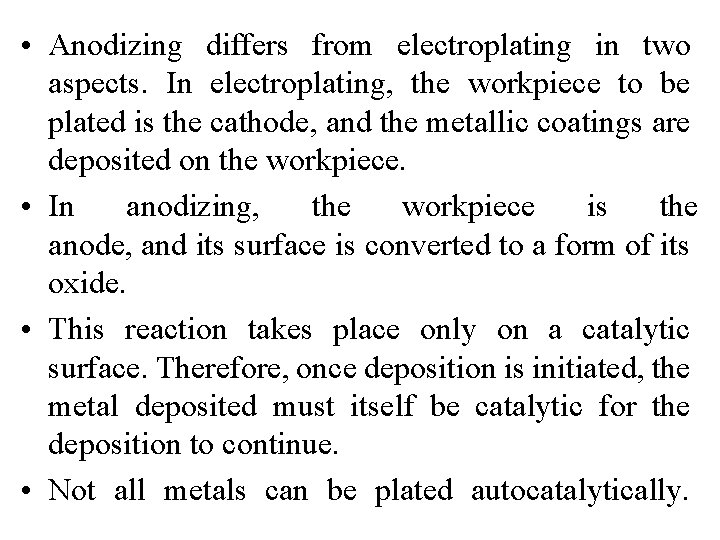
• Anodizing differs from electroplating in two aspects. In electroplating, the workpiece to be plated is the cathode, and the metallic coatings are deposited on the workpiece. • In anodizing, the workpiece is the anode, and its surface is converted to a form of its oxide. • This reaction takes place only on a catalytic surface. Therefore, once deposition is initiated, the metal deposited must itself be catalytic for the deposition to continue. • Not all metals can be plated autocatalytically.
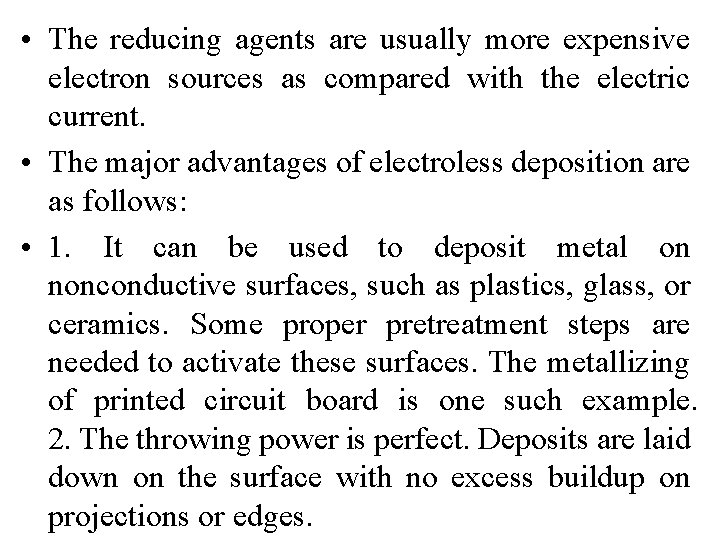
• The reducing agents are usually more expensive electron sources as compared with the electric current. • The major advantages of electroless deposition are as follows: • 1. It can be used to deposit metal on nonconductive surfaces, such as plastics, glass, or ceramics. Some proper pretreatment steps are needed to activate these surfaces. The metallizing of printed circuit board is one such example. 2. The throwing power is perfect. Deposits are laid down on the surface with no excess buildup on projections or edges.
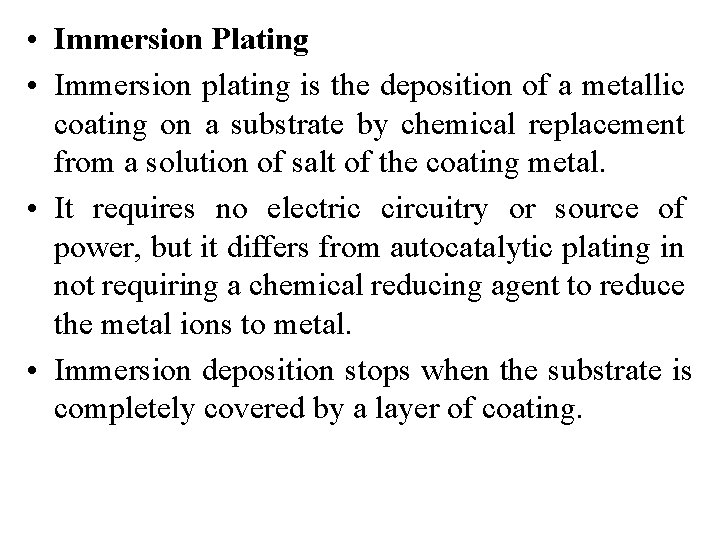
• Immersion Plating • Immersion plating is the deposition of a metallic coating on a substrate by chemical replacement from a solution of salt of the coating metal. • It requires no electric circuitry or source of power, but it differs from autocatalytic plating in not requiring a chemical reducing agent to reduce the metal ions to metal. • Immersion deposition stops when the substrate is completely covered by a layer of coating.
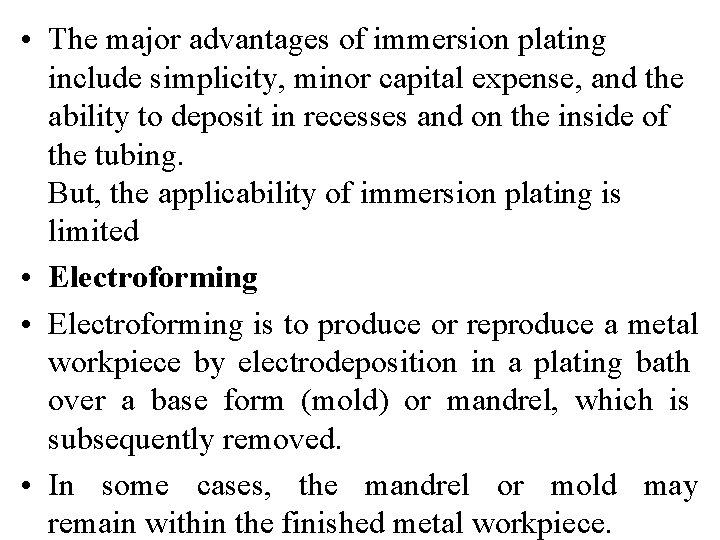
• The major advantages of immersion plating include simplicity, minor capital expense, and the ability to deposit in recesses and on the inside of the tubing. But, the applicability of immersion plating is limited • Electroforming is to produce or reproduce a metal workpiece by electrodeposition in a plating bath over a base form (mold) or mandrel, which is subsequently removed. • In some cases, the mandrel or mold may remain within the finished metal workpiece.
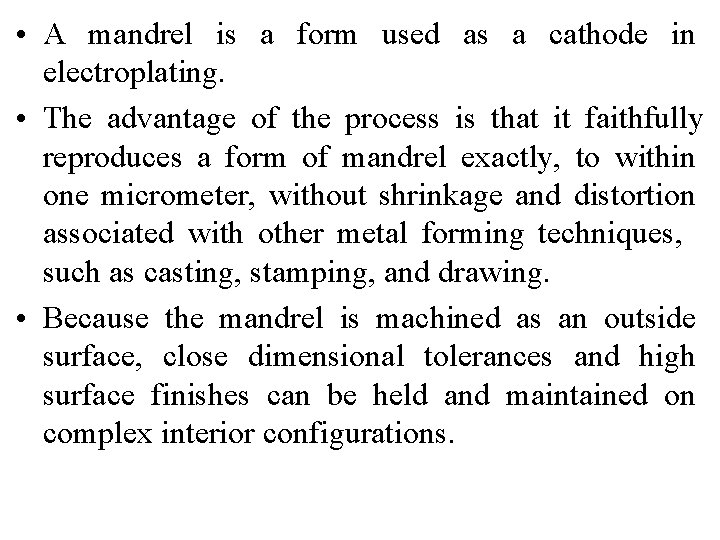
• A mandrel is a form used as a cathode in electroplating. • The advantage of the process is that it faithfully reproduces a form of mandrel exactly, to within one micrometer, without shrinkage and distortion associated with other metal forming techniques, such as casting, stamping, and drawing. • Because the mandrel is machined as an outside surface, close dimensional tolerances and high surface finishes can be held and maintained on complex interior configurations.
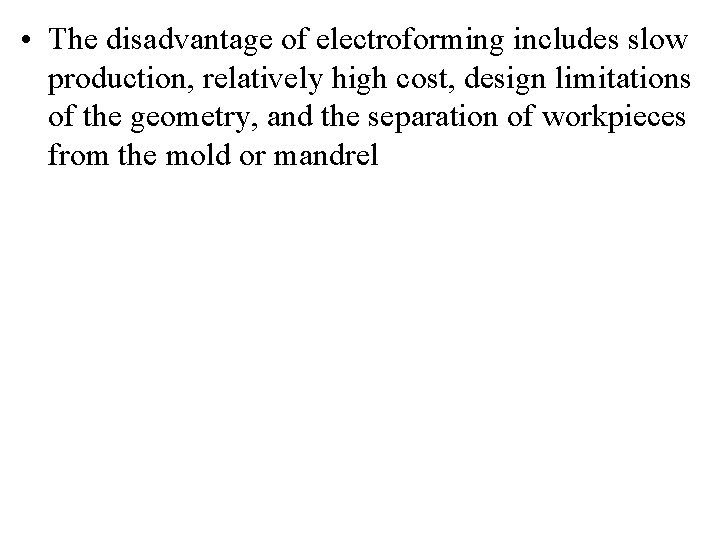
• The disadvantage of electroforming includes slow production, relatively high cost, design limitations of the geometry, and the separation of workpieces from the mold or mandrel
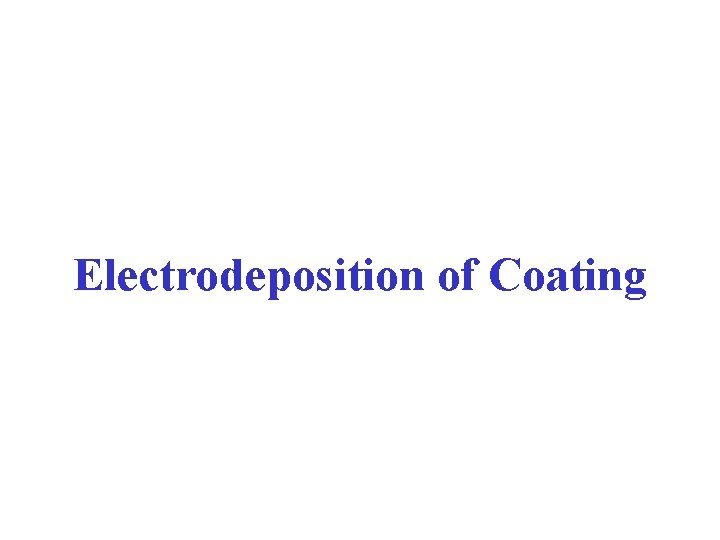
Electrodeposition of Coating
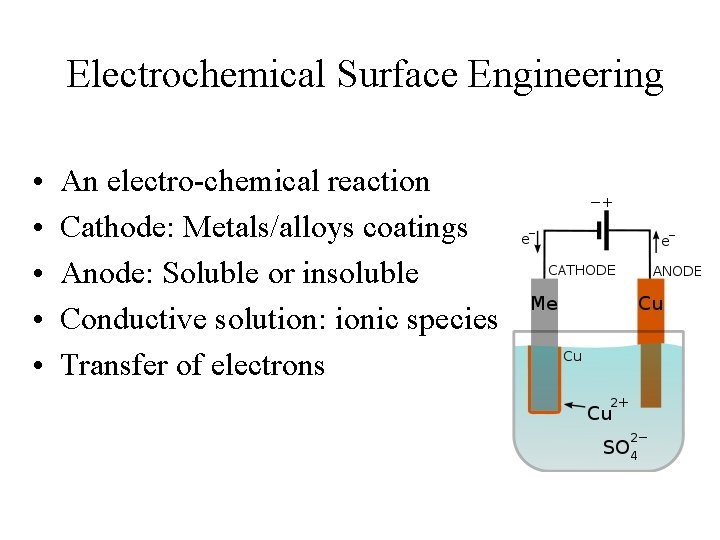
Electrochemical Surface Engineering • • • An electro-chemical reaction Cathode: Metals/alloys coatings Anode: Soluble or insoluble Conductive solution: ionic species Transfer of electrons
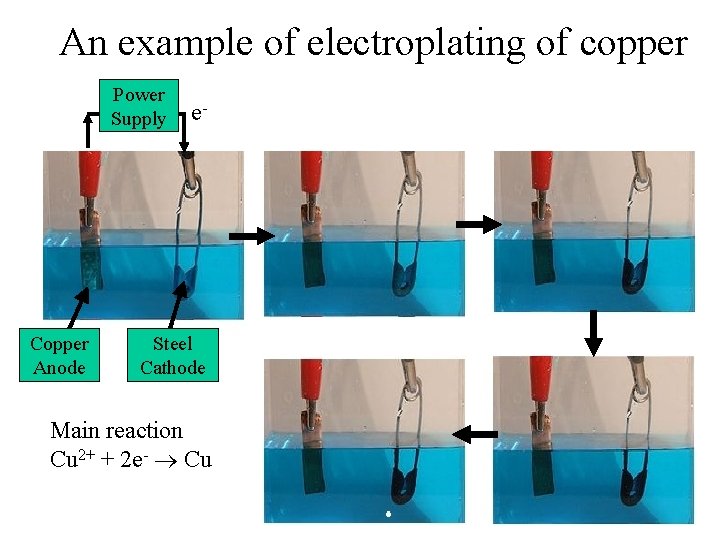
An example of electroplating of copper Power Supply Copper Anode e- Steel Cathode Main reaction Cu 2+ + 2 e- Cu
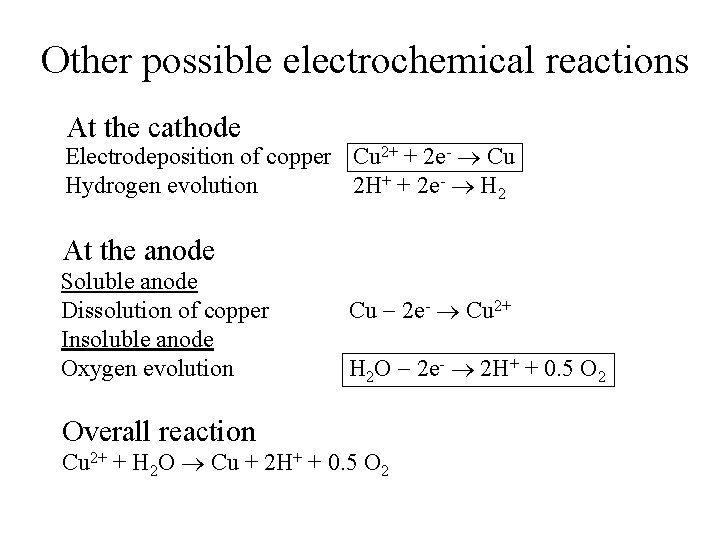
Other possible electrochemical reactions At the cathode Electrodeposition of copper Cu 2+ + 2 e- Cu Hydrogen evolution 2 H+ + 2 e- H 2 At the anode Soluble anode Dissolution of copper Insoluble anode Oxygen evolution Overall reaction Cu 2 e- Cu 2+ H 2 O 2 e- 2 H+ + 0. 5 O 2 Cu 2+ + H 2 O Cu + 2 H+ + 0. 5 O 2
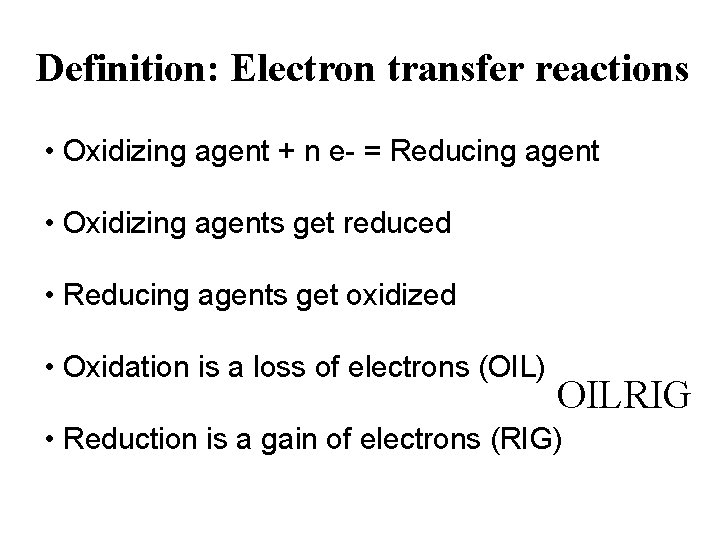
Definition: Electron transfer reactions • Oxidizing agent + n e- = Reducing agent • Oxidizing agents get reduced • Reducing agents get oxidized • Oxidation is a loss of electrons (OIL) OILRIG • Reduction is a gain of electrons (RIG)
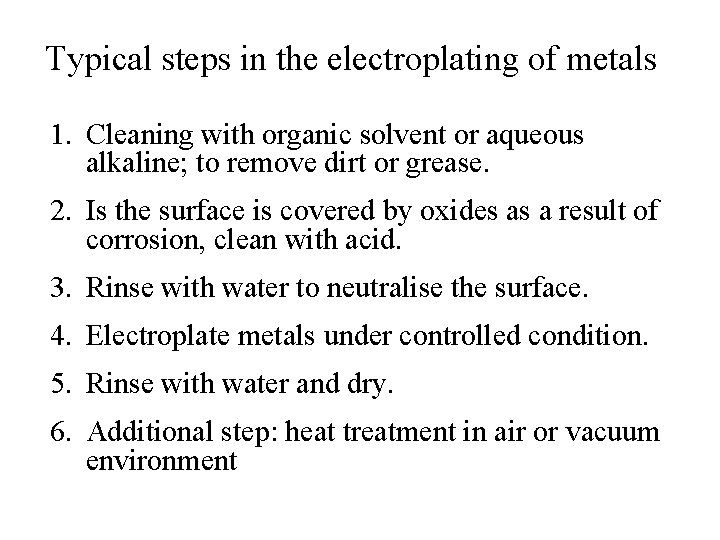
Typical steps in the electroplating of metals 1. Cleaning with organic solvent or aqueous alkaline; to remove dirt or grease. 2. Is the surface is covered by oxides as a result of corrosion, clean with acid. 3. Rinse with water to neutralise the surface. 4. Electroplate metals under controlled condition. 5. Rinse with water and dry. 6. Additional step: heat treatment in air or vacuum environment
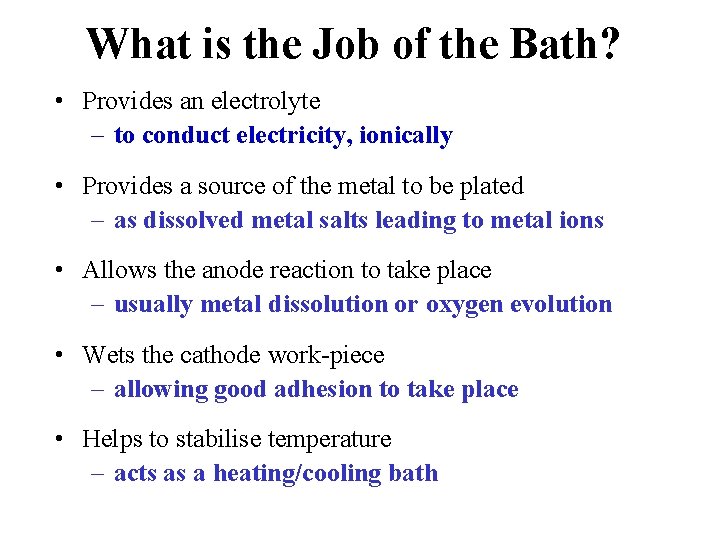
What is the Job of the Bath? • Provides an electrolyte – to conduct electricity, ionically • Provides a source of the metal to be plated – as dissolved metal salts leading to metal ions • Allows the anode reaction to take place – usually metal dissolution or oxygen evolution • Wets the cathode work-piece – allowing good adhesion to take place • Helps to stabilise temperature – acts as a heating/cooling bath
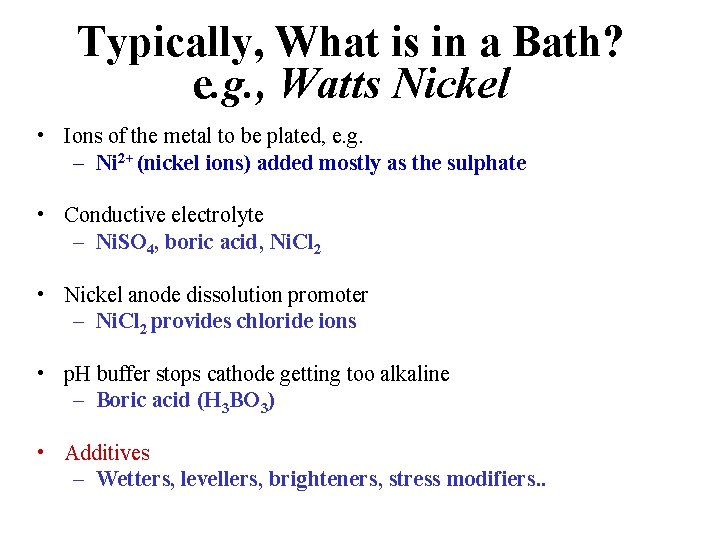
Typically, What is in a Bath? e. g. , Watts Nickel • Ions of the metal to be plated, e. g. – Ni 2+ (nickel ions) added mostly as the sulphate • Conductive electrolyte – Ni. SO 4, boric acid, Ni. Cl 2 • Nickel anode dissolution promoter – Ni. Cl 2 provides chloride ions • p. H buffer stops cathode getting too alkaline – Boric acid (H 3 BO 3) • Additives – Wetters, levellers, brighteners, stress modifiers. .
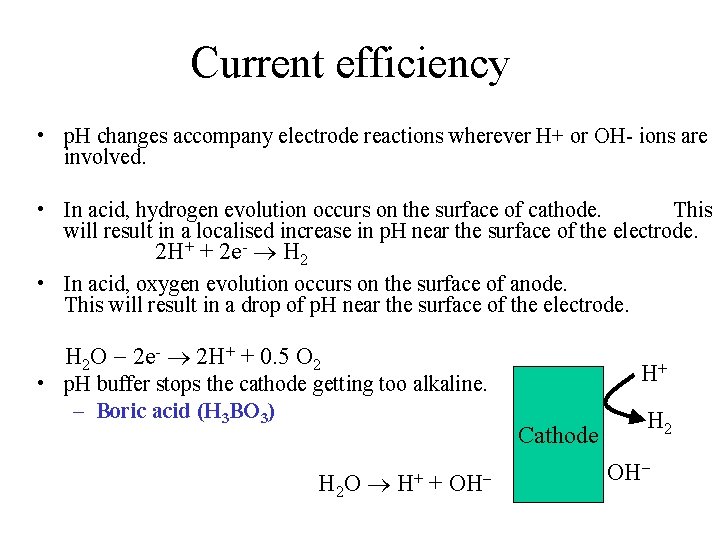
Current efficiency • p. H changes accompany electrode reactions wherever H+ or OH- ions are involved. • In acid, hydrogen evolution occurs on the surface of cathode. This will result in a localised increase in p. H near the surface of the electrode. 2 H+ + 2 e- H 2 • In acid, oxygen evolution occurs on the surface of anode. This will result in a drop of p. H near the surface of the electrode. H 2 O 2 e- 2 H+ + 0. 5 O 2 • p. H buffer stops the cathode getting too alkaline. – Boric acid (H 3 BO 3) H 2 O H+ + OH H+ Cathode H 2 OH
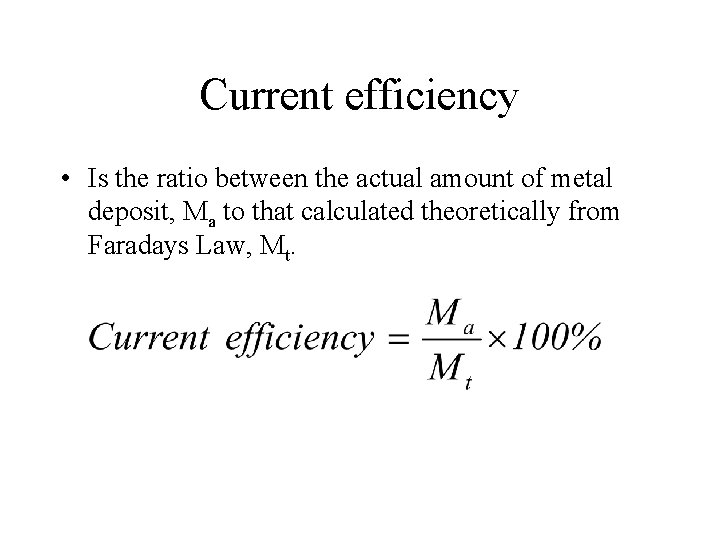
Current efficiency • Is the ratio between the actual amount of metal deposit, Ma to that calculated theoretically from Faradays Law, Mt.
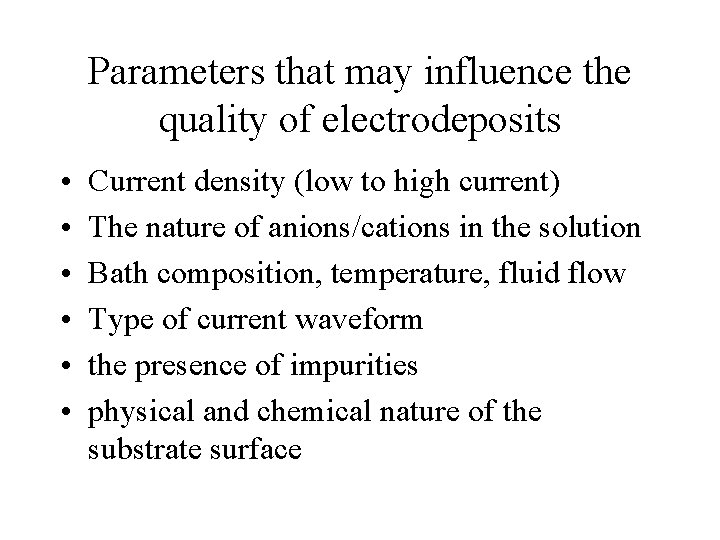
Parameters that may influence the quality of electrodeposits • • • Current density (low to high current) The nature of anions/cations in the solution Bath composition, temperature, fluid flow Type of current waveform the presence of impurities physical and chemical nature of the substrate surface
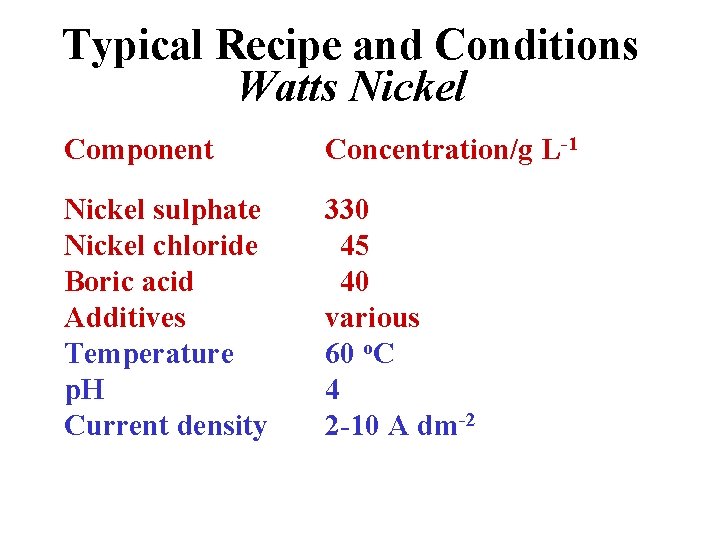
Typical Recipe and Conditions Watts Nickel Component Concentration/g L-1 Nickel sulphate Nickel chloride Boric acid Additives Temperature p. H Current density 330 45 40 various 60 o. C 4 2 -10 A dm-2
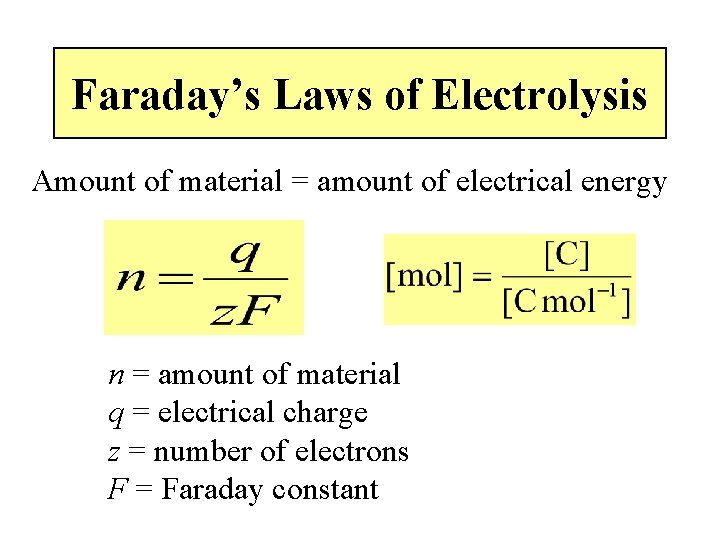
Faraday’s Laws of Electrolysis Amount of material = amount of electrical energy n = amount of material q = electrical charge z = number of electrons F = Faraday constant
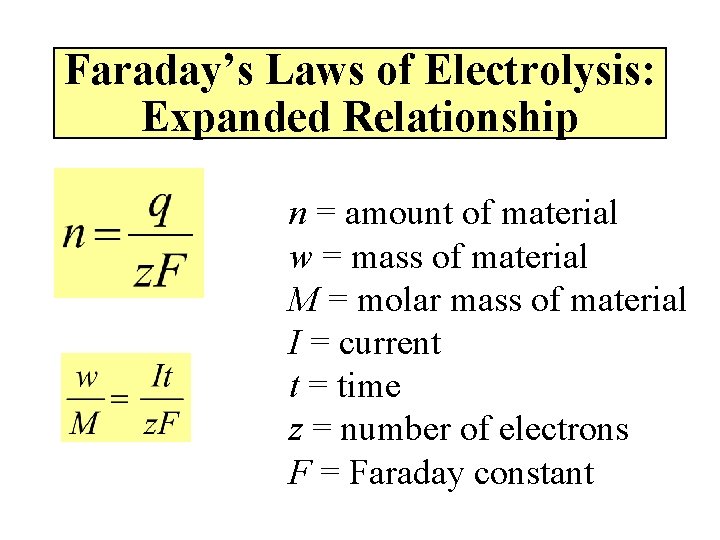
Faraday’s Laws of Electrolysis: Expanded Relationship n = amount of material w = mass of material M = molar mass of material I = current t = time z = number of electrons F = Faraday constant
![Current Current density Surface area j current density m A cm2 I Current, Current density, Surface area j = current density [m. A cm-2] I =](https://slidetodoc.com/presentation_image_h2/78f3b1ea4895bc2c5935e6be093fcd7a/image-104.jpg)
Current, Current density, Surface area j = current density [m. A cm-2] I = current [A] A = surface area of the electrode [cm 2] jelectroplate = electroplating current density (metal electroplate) jcorrosion = corrosion current density (metal corrosion/dissolution)
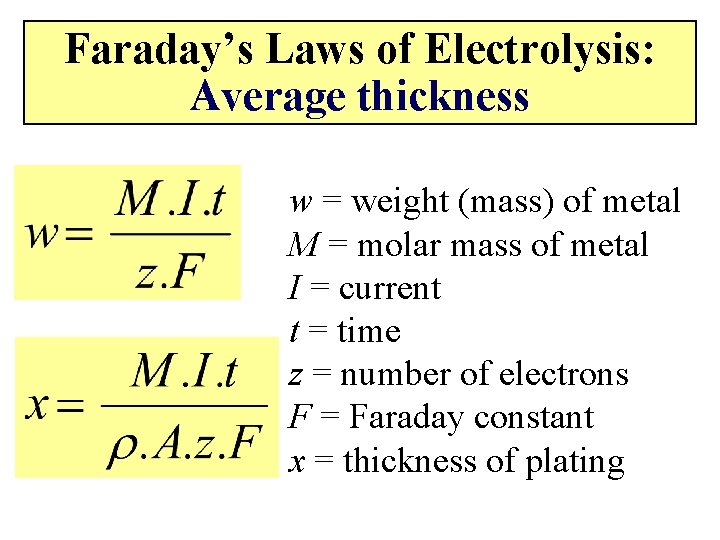
Faraday’s Laws of Electrolysis: Average thickness w = weight (mass) of metal M = molar mass of metal I = current t = time z = number of electrons F = Faraday constant x = thickness of plating
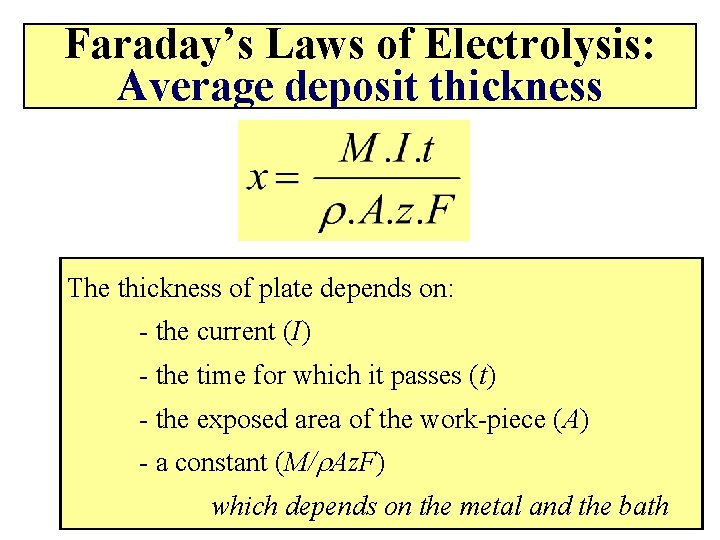
Faraday’s Laws of Electrolysis: Average deposit thickness The thickness of plate depends on: - the current (I) - the time for which it passes (t) - the exposed area of the work-piece (A) - a constant (M/r. Az. F) which depends on the metal and the bath
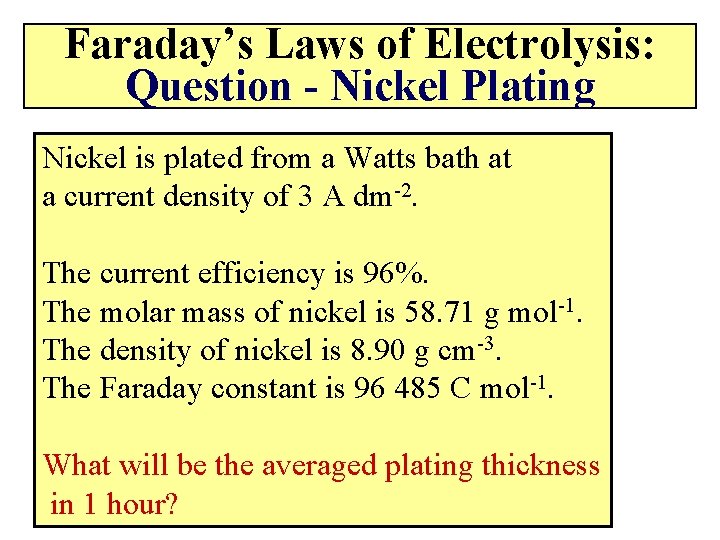
Faraday’s Laws of Electrolysis: Question - Nickel Plating Nickel is plated from a Watts bath at a current density of 3 A dm-2. The current efficiency is 96%. The molar mass of nickel is 58. 71 g mol-1. The density of nickel is 8. 90 g cm-3. The Faraday constant is 96 485 C mol-1. What will be the averaged plating thickness in 1 hour?
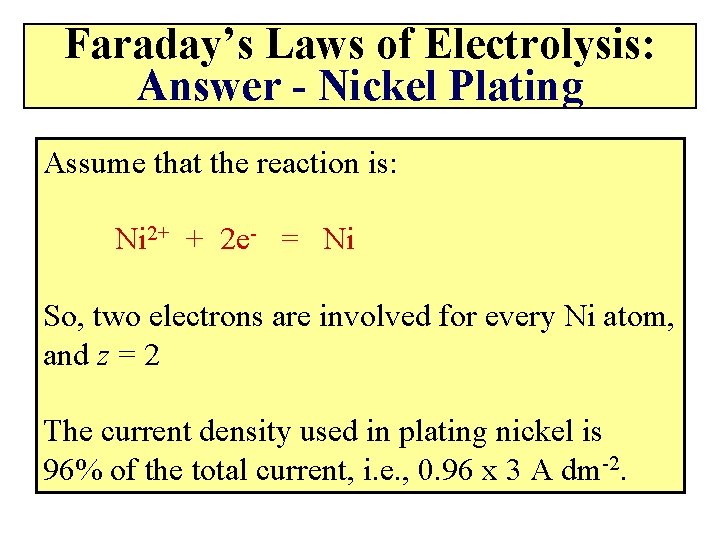
Faraday’s Laws of Electrolysis: Answer - Nickel Plating Assume that the reaction is: Ni 2+ + 2 e- = Ni So, two electrons are involved for every Ni atom, and z = 2 The current density used in plating nickel is 96% of the total current, i. e. , 0. 96 x 3 A dm-2.
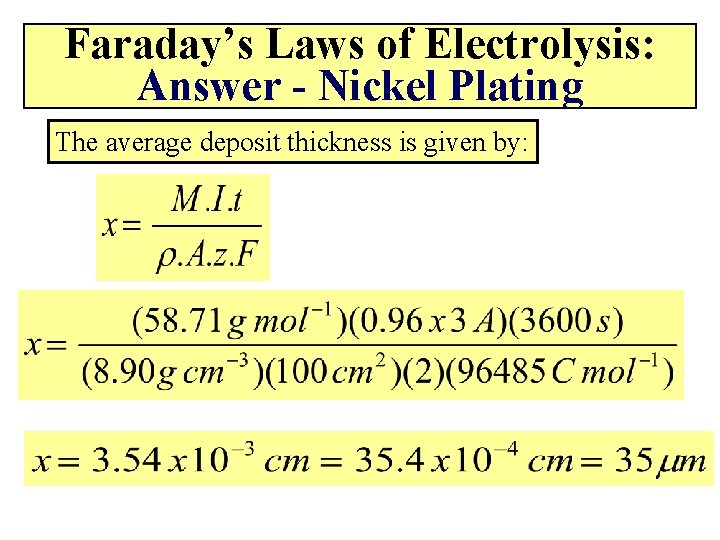
Faraday’s Laws of Electrolysis: Answer - Nickel Plating The average deposit thickness is given by:
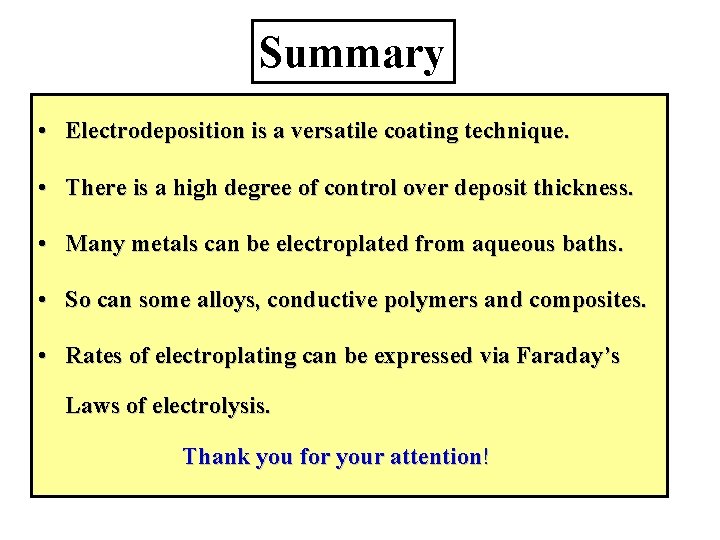
Summary • Electrodeposition is a versatile coating technique. • There is a high degree of control over deposit thickness. • Many metals can be electroplated from aqueous baths. • So can some alloys, conductive polymers and composites. • Rates of electroplating can be expressed via Faraday’s Laws of electrolysis. Thank you for your attention!
Cathode anode standard reduction potential
Difference between galvanic cell and electrolytic cell
Lithium ion battery reaction equation
Equilibrium constant formula electrochemistry
Half redox reaction
Voltaic and galvanic cells
Standard reduction potential table
Electrolytic cell khan academy
Leclanche cell anode and cathode reactions
Why are galvanic cells spontaneous
Use the tabulated half-cell potentials to calculate
Is anode positive or negative
Nernst equation
Parts of a galvanic cell
Image of galvanic cell
How does the galvanic body spa work
Line notation electrochemistry
Development of paranasal sinuses
Red blood cells and white blood cells difference
Plant vs animal cells venn diagram
Masses of cells form and steal nutrients from healthy cells
Epsp vs ipsp
Source of bioelectric potential isin nature.
Graded potential vs action potential
Define graded potential
Postsynaptic potentials
Lienard-wiechert potentials
Eθcell
What are electrical synapses
Explain helmholtz electrical double layer
Maxwell relation
Measurable quantities
Enthalpy vs internal energy
Electromagnetic potentials
Electric potential parallel plate capacitor
Loop of henle
Parafollicular cells vs follicular cells
Somatic vs gamete
Why dna is more stable than rna
Prokaryotic cell vs eukaryotic cell
Prokaryotes vs eukaryotes venn diagram
Organelle trail
Younger cells cuboidal older cells flattened
What cell type
Staphylococcus aureus prokaryotic or eukaryotic
Chapter 8 cellular reproduction cells from cells
Cells cells they're made of organelles meme
Galvanic burns
Aluminium designation
Galvanic body spa funciona realmente
Cuplaj galvanic
Electrochemical theory of corrosion
Galvanic body spa benefits
Cpi chemical process industry
Ageloc galvanic body trio
Collections of specialized cells and cell products
Standard error of mean
Power dynamics of the standard language
Definition of standard costing
Faraday's constant
Transport number chemistry
Electrochemistry tutorial
Ap chemistry chapter 18 electrochemistry test
What is electrochemistry in chemistry
Electrochemistry balancing equations
Electrochemistry stoichiometry
Introduction to electrochemistry
Basic electrochemistry
Complex redox reactions
Ap chem electrochemistry review
Electrochemistry ap chemistry
Cell chapter 21
Equilibrium constant formula electrochemistry
Interfacial method in electrochemistry
Electrochemistry eds
Fundamentals of electrochemistry
What is electrochemistry
What is electrochemistry in chemistry
Diagonal rule electrochemistry
Polarization in electrochemistry
Electrochemistry lesson
Slidesdgo
Electrochemistry ap chemistry
Mass transport electrochemistry
Electroanalytical chemistry definition
Fundamentals of electrochemistry
What is electrochemistry
Diagonal rule electrochemistry
Ir drop in electrochemistry
Electrochemistry balancing equations
Analytical electrochemistry
Voltaic aim
Concentration cell
Electrochemistry
Electrons flow from anode to cathode
Germ cell vs somatic cells
Mbk berkefungsian rendah
Denuding tower
Prokaryotic and eukaryotic cells
Venn diagram animal and plant cells
Plant cell structure
Animal and plant cell diagram
Primary and secondary cells
Whats the difference between plant and animal cells
Section 10-2 cell division
Prokaryotic cell and eukaryotic cell
The scientist mathias schleiden studied _______ in ______.
Idealized plant cell
Walker cell and hadley cell
Cell cycle and cell division
Cell cycle chart