Transport phenomena in electrochemical systems Charge and mass
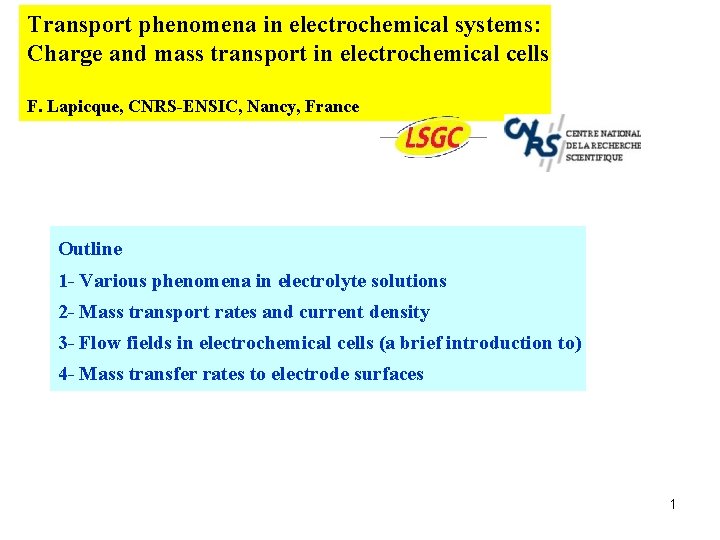
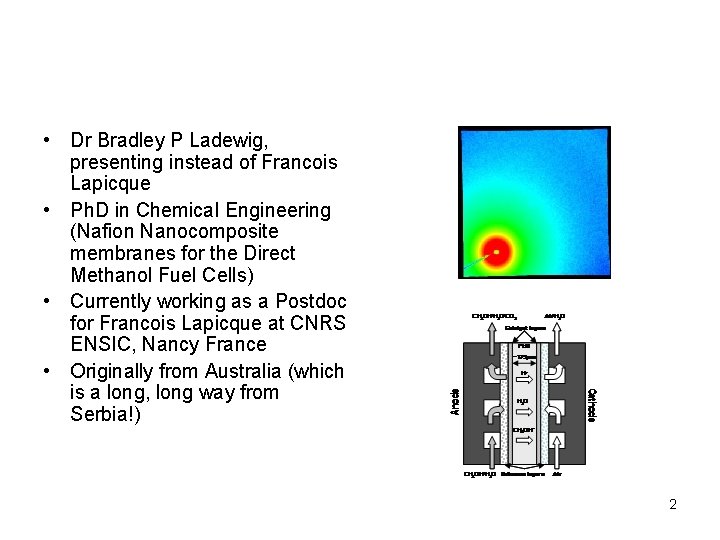
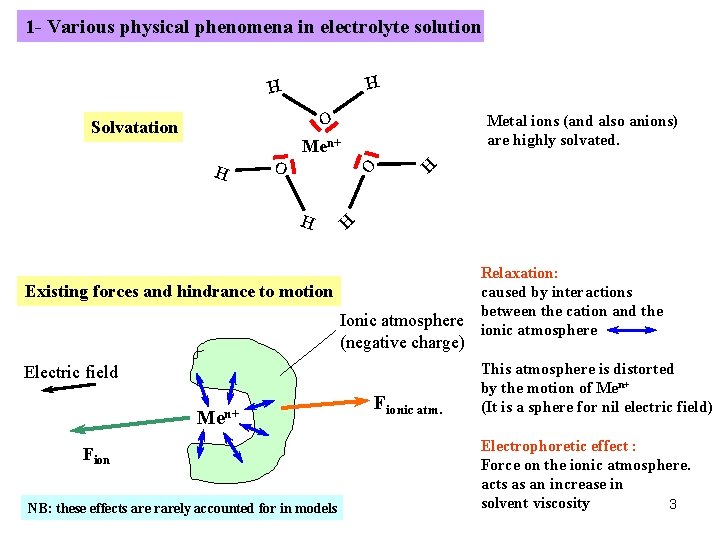
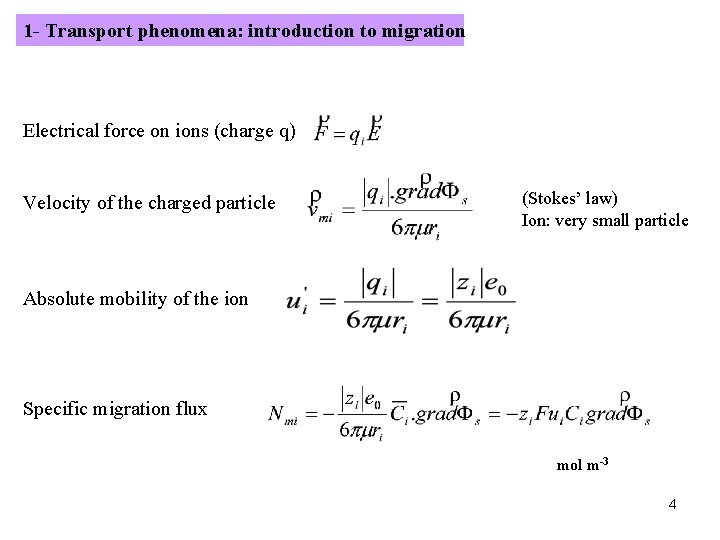
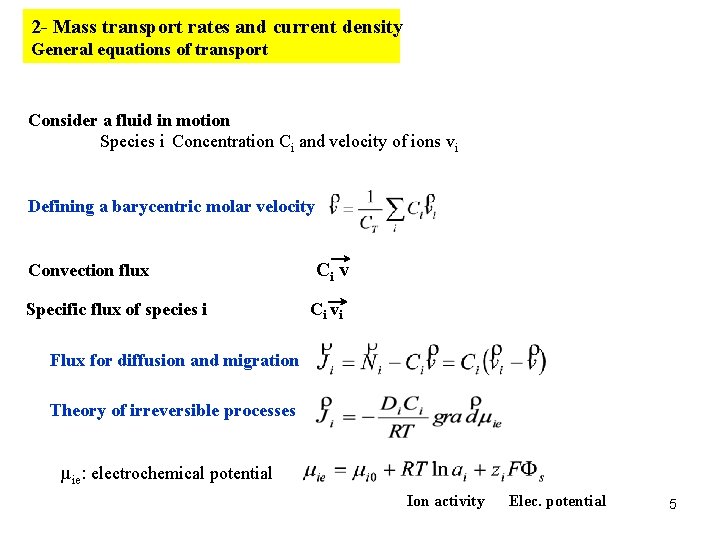
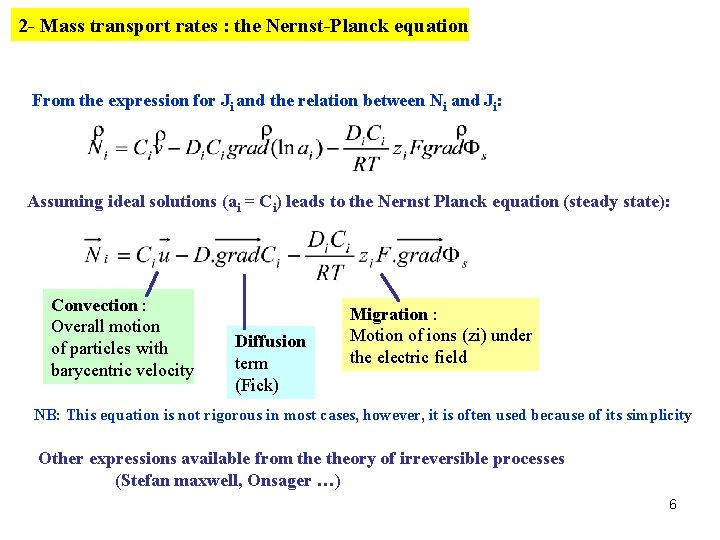
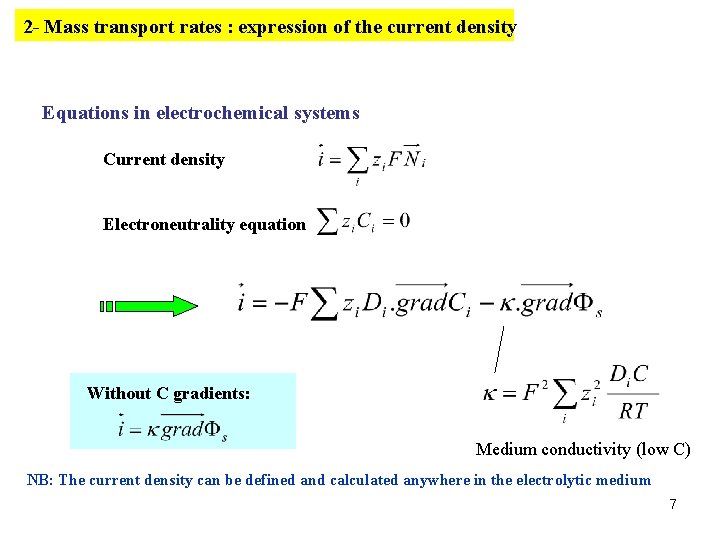
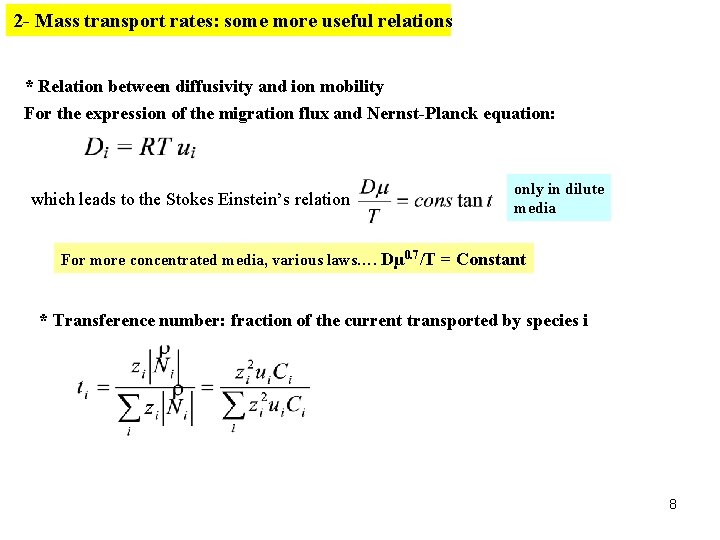
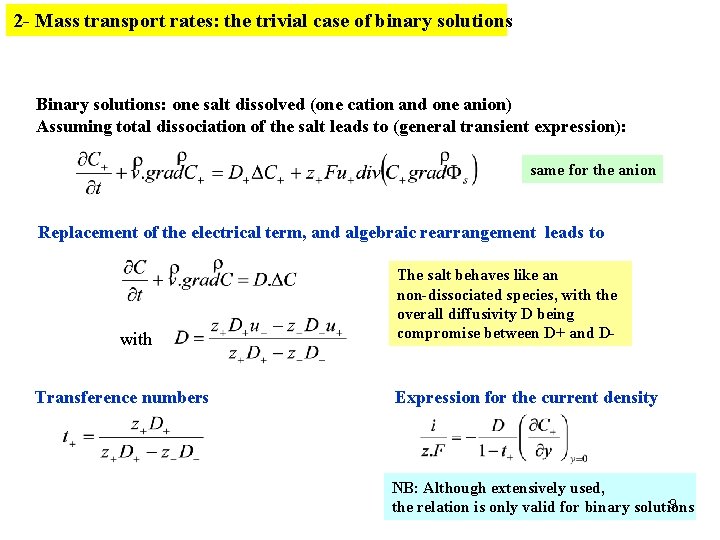
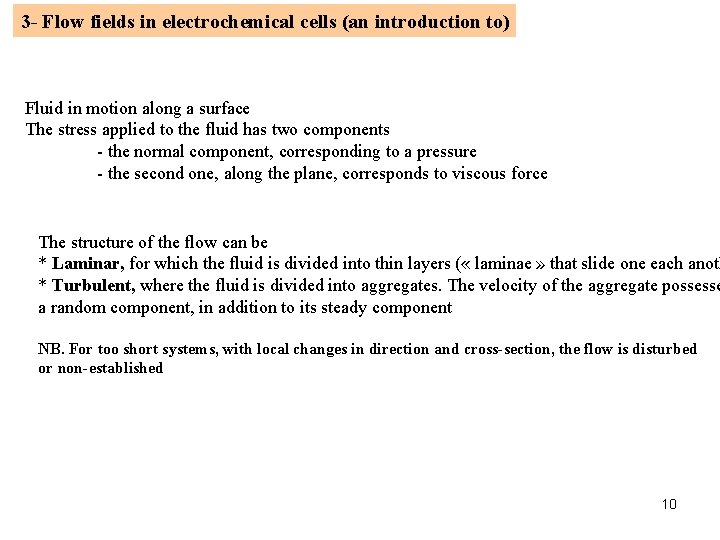
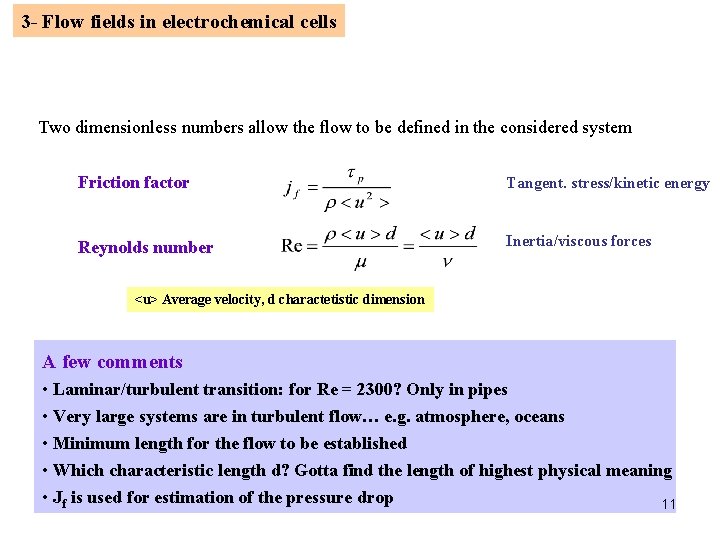
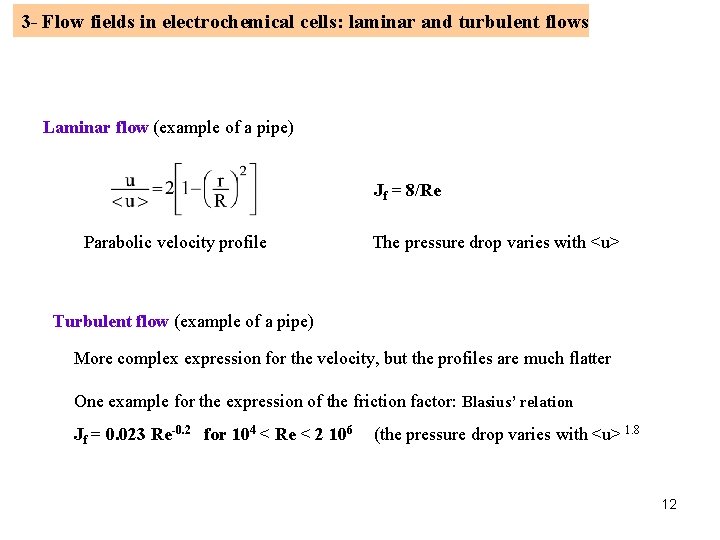
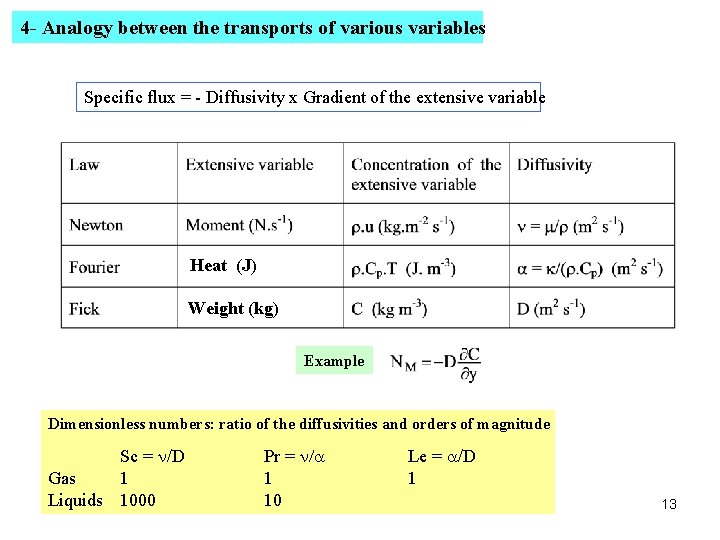
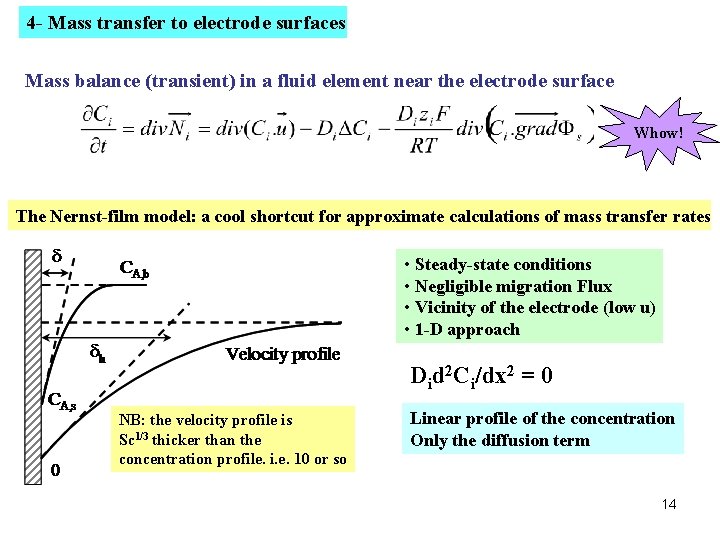
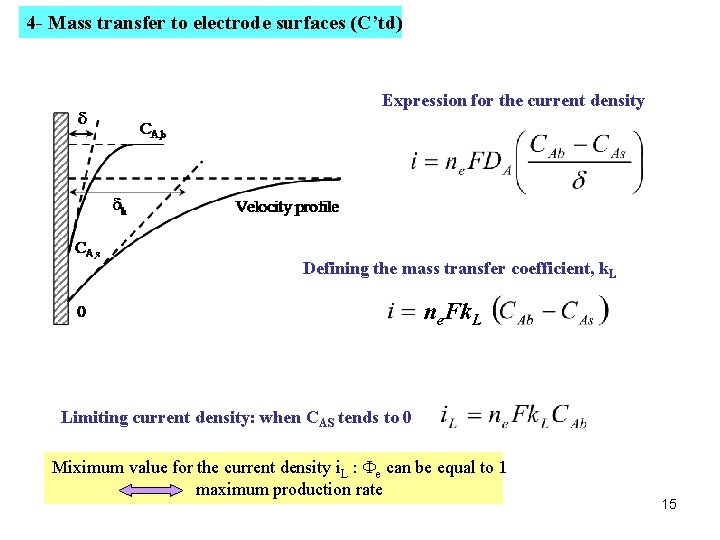
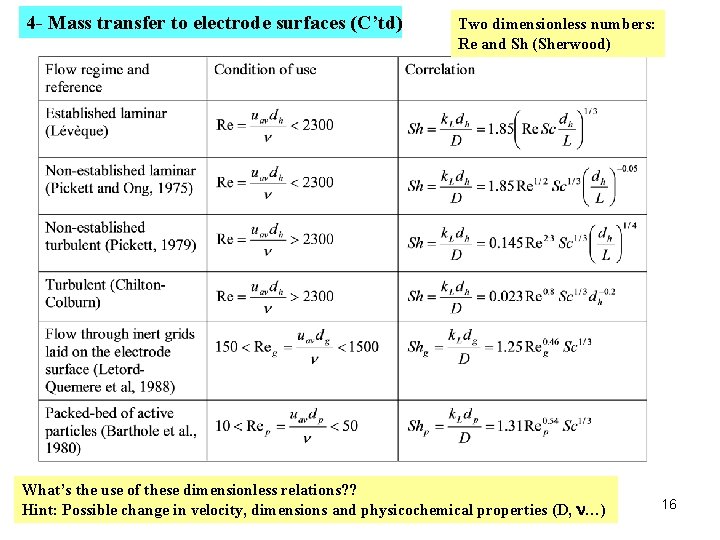
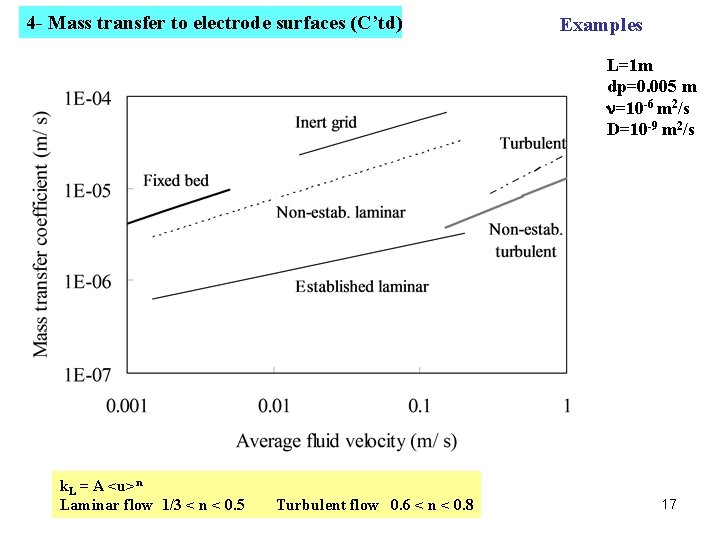
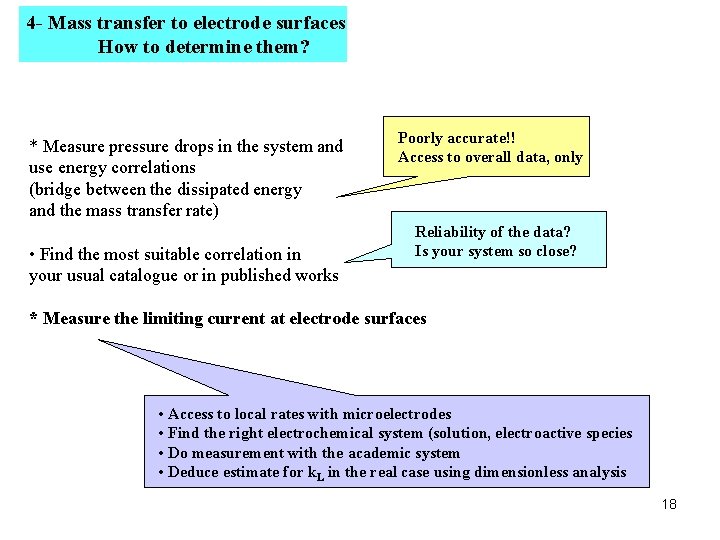
- Slides: 18
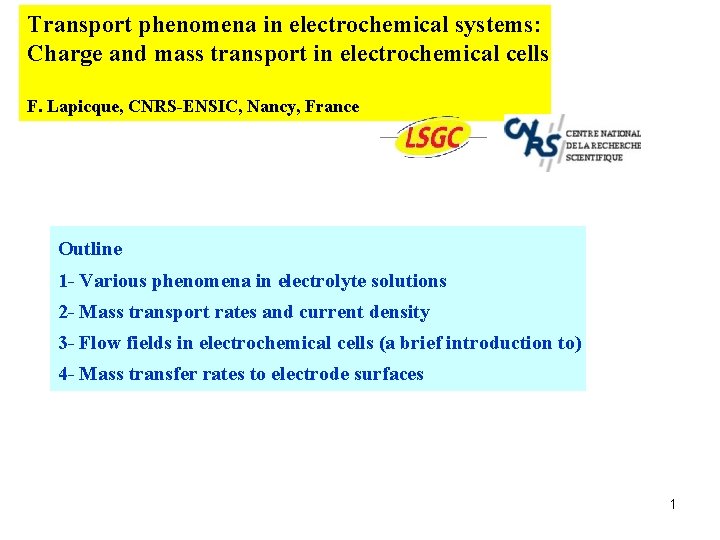
Transport phenomena in electrochemical systems: Charge and mass transport in electrochemical cells F. Lapicque, CNRS-ENSIC, Nancy, France Outline 1 - Various phenomena in electrolyte solutions 2 - Mass transport rates and current density 3 - Flow fields in electrochemical cells (a brief introduction to) 4 - Mass transfer rates to electrode surfaces 1
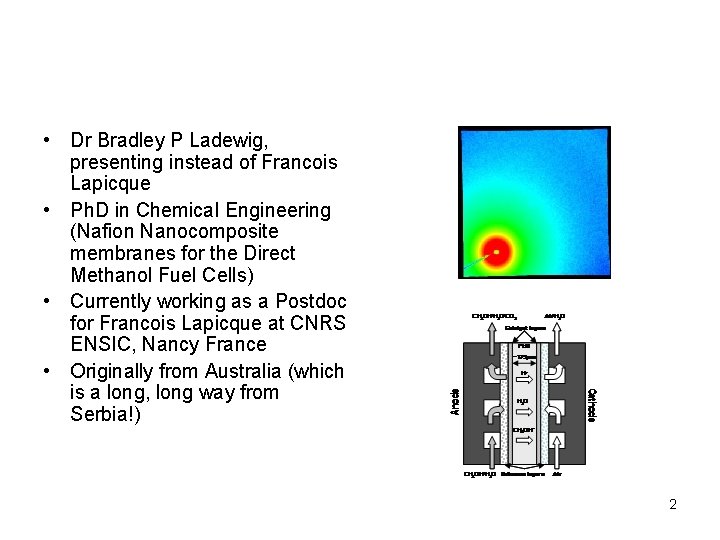
• Dr Bradley P Ladewig, presenting instead of Francois Lapicque • Ph. D in Chemical Engineering (Nafion Nanocomposite membranes for the Direct Methanol Fuel Cells) • Currently working as a Postdoc for Francois Lapicque at CNRS ENSIC, Nancy France • Originally from Australia (which is a long, long way from Serbia!) 2
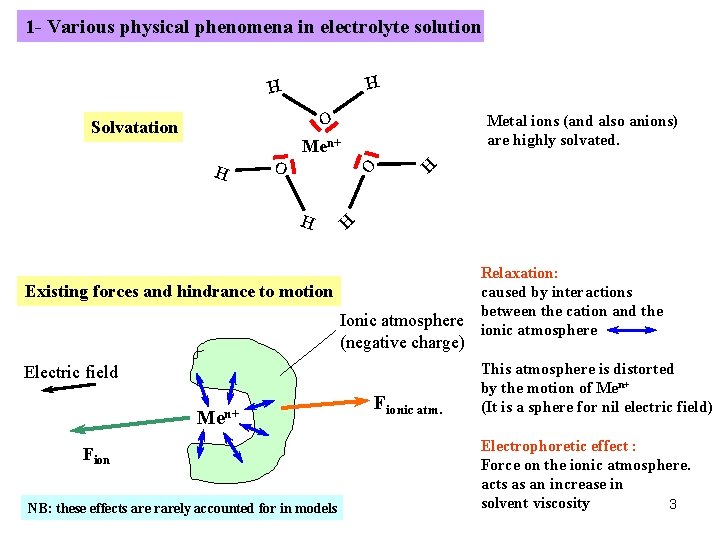
1 - Various physical phenomena in electrolyte solution H H O Men+ O H Metal ions (and also anions) are highly solvated. H Solvatation Existing forces and hindrance to motion Ionic atmosphere (negative charge) Electric field Men+ Fion NB: these effects are rarely accounted for in models Fionic atm. Relaxation: caused by interactions between the cation and the ionic atmosphere This atmosphere is distorted by the motion of Men+ (It is a sphere for nil electric field) Electrophoretic effect : Force on the ionic atmosphere. acts as an increase in solvent viscosity 3
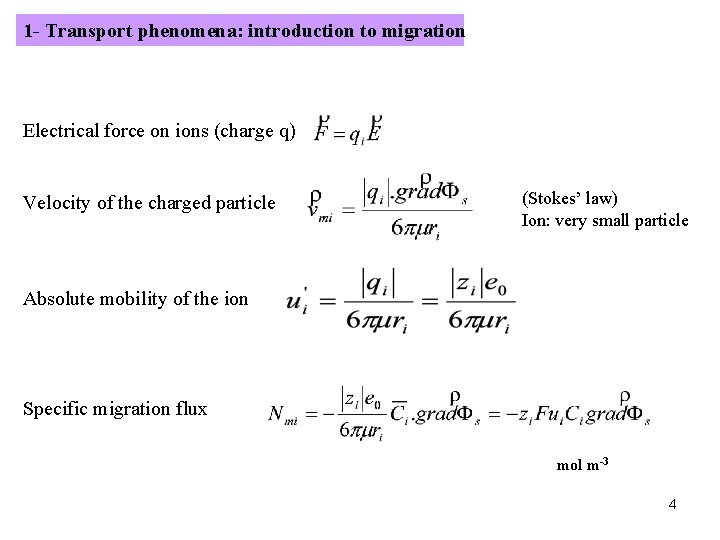
1 - Transport phenomena: introduction to migration Electrical force on ions (charge q) Velocity of the charged particle (Stokes’ law) Ion: very small particle Absolute mobility of the ion Specific migration flux mol m-3 4
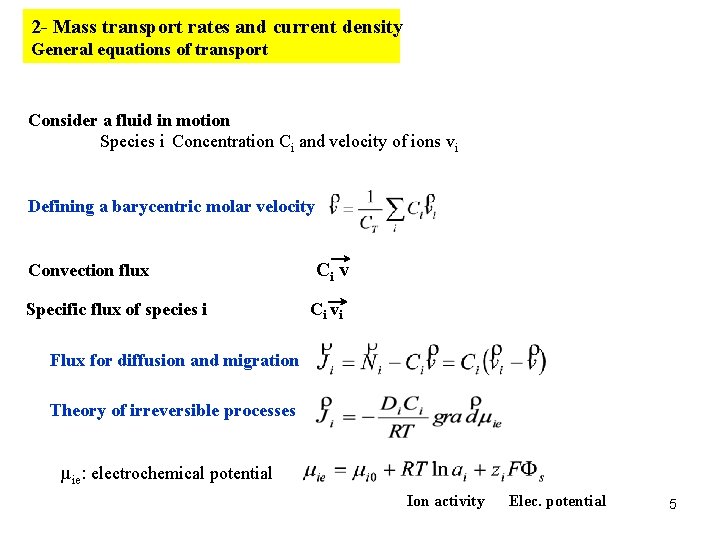
2 - Mass transport rates and current density General equations of transport Consider a fluid in motion Species i Concentration Ci and velocity of ions vi Defining a barycentric molar velocity Convection flux Specific flux of species i Ci vi Flux for diffusion and migration Theory of irreversible processes µie: electrochemical potential Ion activity Elec. potential 5
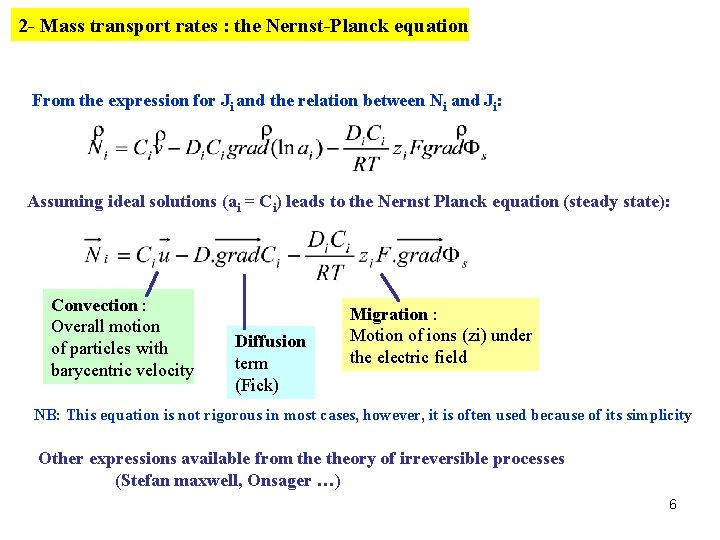
2 - Mass transport rates : the Nernst-Planck equation From the expression for Ji and the relation between Ni and Ji: Assuming ideal solutions (ai = Ci) leads to the Nernst Planck equation (steady state): Convection : Overall motion of particles with barycentric velocity Diffusion term (Fick) Migration : Motion of ions (zi) under the electric field NB: This equation is not rigorous in most cases, however, it is often used because of its simplicity Other expressions available from theory of irreversible processes (Stefan maxwell, Onsager …) 6
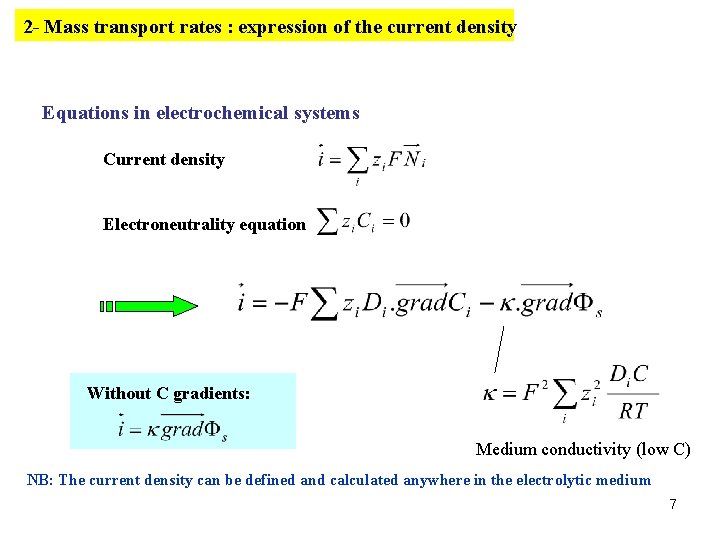
2 - Mass transport rates : expression of the current density Equations in electrochemical systems Current density Electroneutrality equation Without C gradients: Medium conductivity (low C) NB: The current density can be defined and calculated anywhere in the electrolytic medium 7
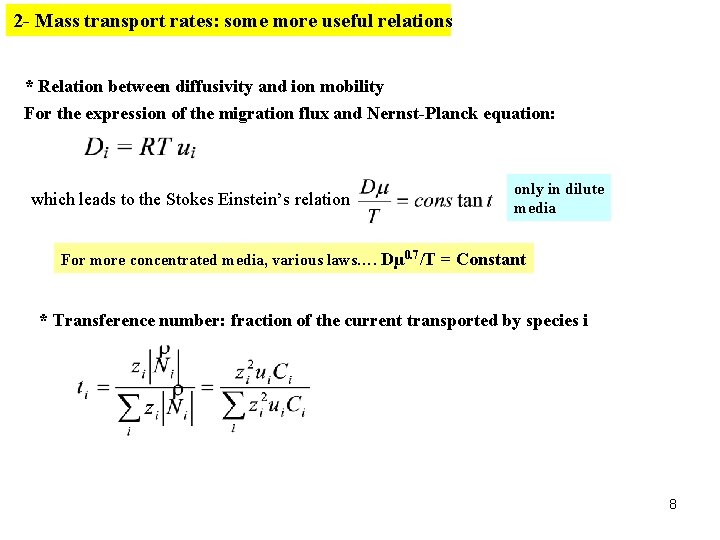
2 - Mass transport rates: some more useful relations * Relation between diffusivity and ion mobility For the expression of the migration flux and Nernst-Planck equation: which leads to the Stokes Einstein’s relation only in dilute media For more concentrated media, various laws…. Dµ 0. 7/T = Constant * Transference number: fraction of the current transported by species i 8
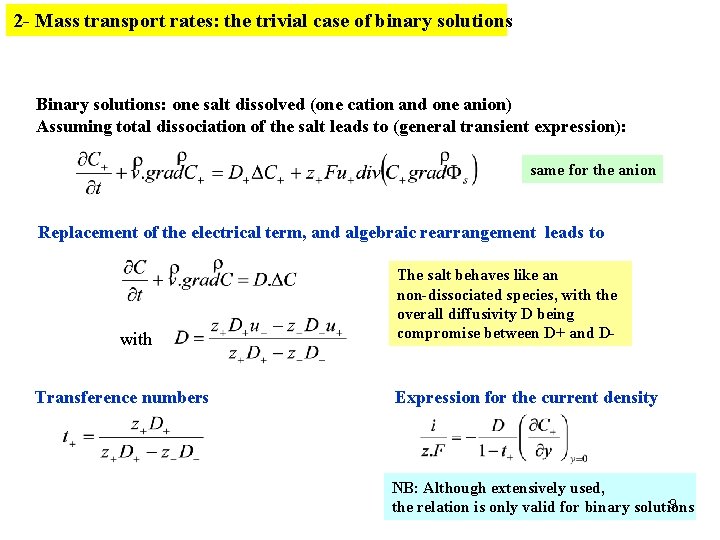
2 - Mass transport rates: the trivial case of binary solutions Binary solutions: one salt dissolved (one cation and one anion) Assuming total dissociation of the salt leads to (general transient expression): same for the anion Replacement of the electrical term, and algebraic rearrangement leads to with Transference numbers The salt behaves like an non-dissociated species, with the overall diffusivity D being compromise between D+ and D- Expression for the current density NB: Although extensively used, 9 the relation is only valid for binary solutions
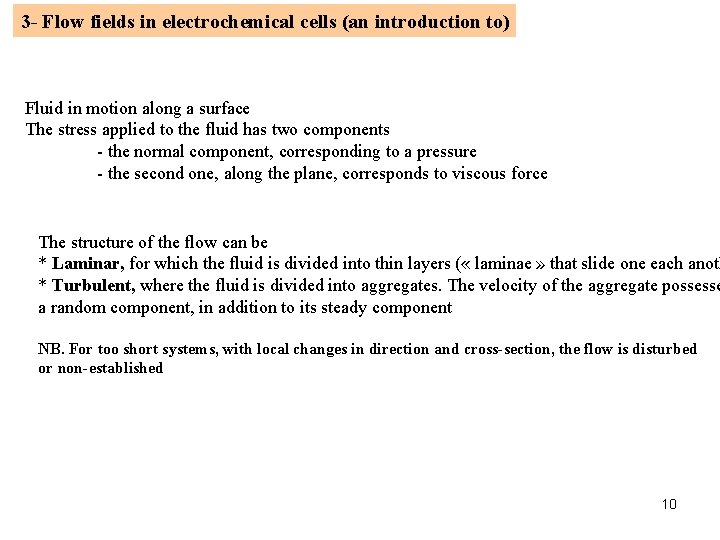
3 - Flow fields in electrochemical cells (an introduction to) Fluid in motion along a surface The stress applied to the fluid has two components - the normal component, corresponding to a pressure - the second one, along the plane, corresponds to viscous force The structure of the flow can be * Laminar, for which the fluid is divided into thin layers ( « laminae » that slide one each anoth * Turbulent, where the fluid is divided into aggregates. The velocity of the aggregate possesse a random component, in addition to its steady component NB. For too short systems, with local changes in direction and cross-section, the flow is disturbed or non-established 10
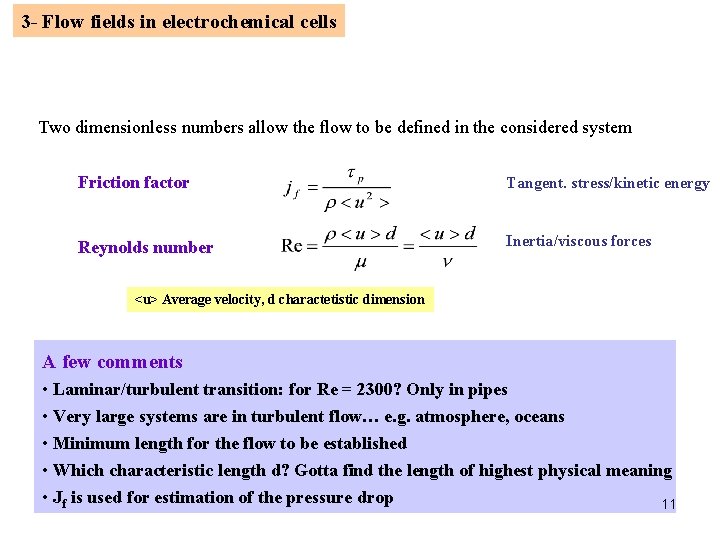
3 - Flow fields in electrochemical cells Two dimensionless numbers allow the flow to be defined in the considered system Friction factor Tangent. stress/kinetic energy Reynolds number Inertia/viscous forces <u> Average velocity, d charactetistic dimension A few comments • Laminar/turbulent transition: for Re = 2300? Only in pipes • Very large systems are in turbulent flow… e. g. atmosphere, oceans • Minimum length for the flow to be established • Which characteristic length d? Gotta find the length of highest physical meaning • Jf is used for estimation of the pressure drop 11
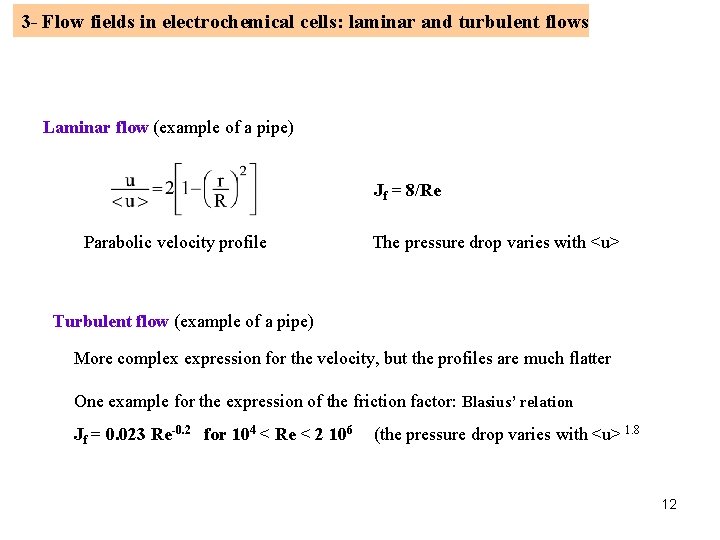
3 - Flow fields in electrochemical cells: laminar and turbulent flows Laminar flow (example of a pipe) Jf = 8/Re Parabolic velocity profile The pressure drop varies with <u> Turbulent flow (example of a pipe) More complex expression for the velocity, but the profiles are much flatter One example for the expression of the friction factor: Blasius’ relation Jf = 0. 023 Re-0. 2 for 104 < Re < 2 106 (the pressure drop varies with <u> 1. 8 12
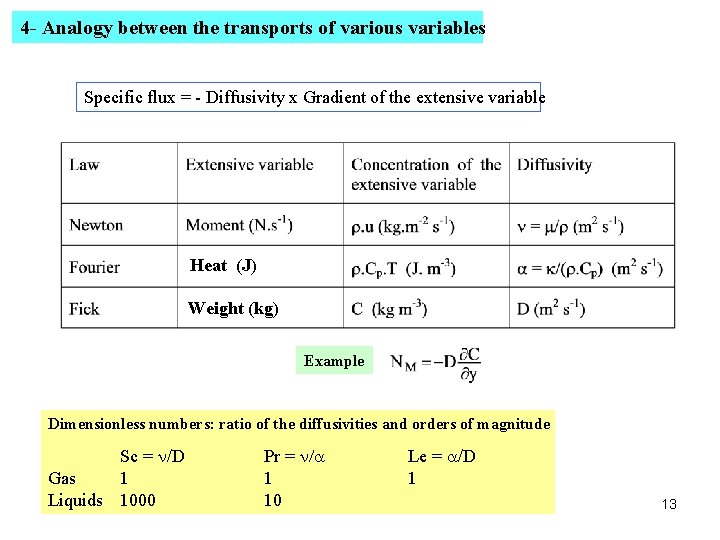
4 - Analogy between the transports of various variables Specific flux = - Diffusivity x Gradient of the extensive variable Heat (J) Weight (kg) Example Dimensionless numbers: ratio of the diffusivities and orders of magnitude Sc = n/D Gas 1 Liquids 1000 Pr = n/a 1 10 Le = a/D 1 13
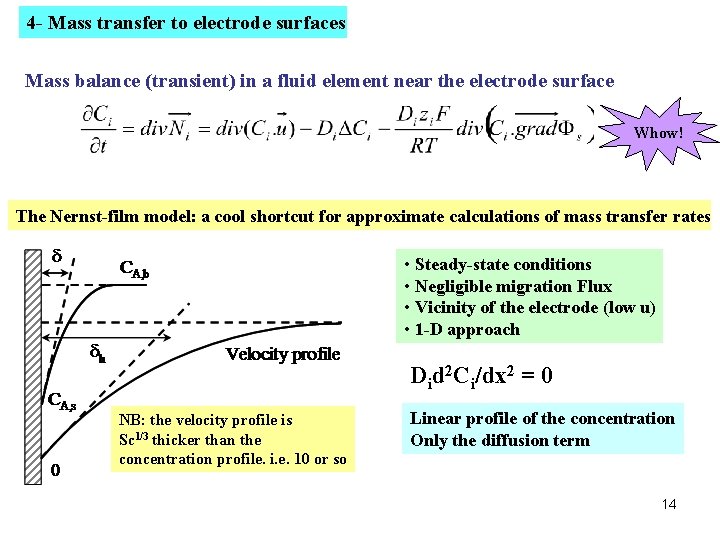
4 - Mass transfer to electrode surfaces Mass balance (transient) in a fluid element near the electrode surface Whow! The Nernst-film model: a cool shortcut for approximate calculations of mass transfer rates • Steady-state conditions • Negligible migration Flux • Vicinity of the electrode (low u) • 1 -D approach Did 2 Ci/dx 2 = 0 NB: the velocity profile is Sc 1/3 thicker than the concentration profile. i. e. 10 or so Linear profile of the concentration Only the diffusion term 14
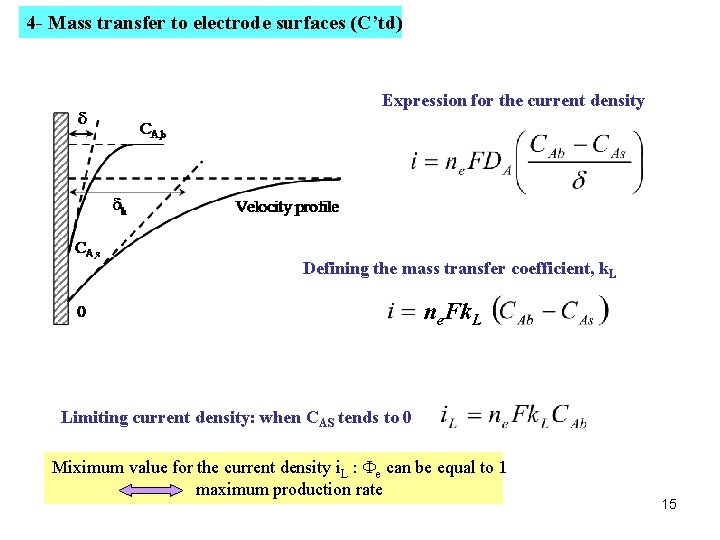
4 - Mass transfer to electrode surfaces (C’td) Expression for the current density Defining the mass transfer coefficient, k. L ne. Fk. L Limiting current density: when CAS tends to 0 Miximum value for the current density i. L : Fe can be equal to 1 maximum production rate 15
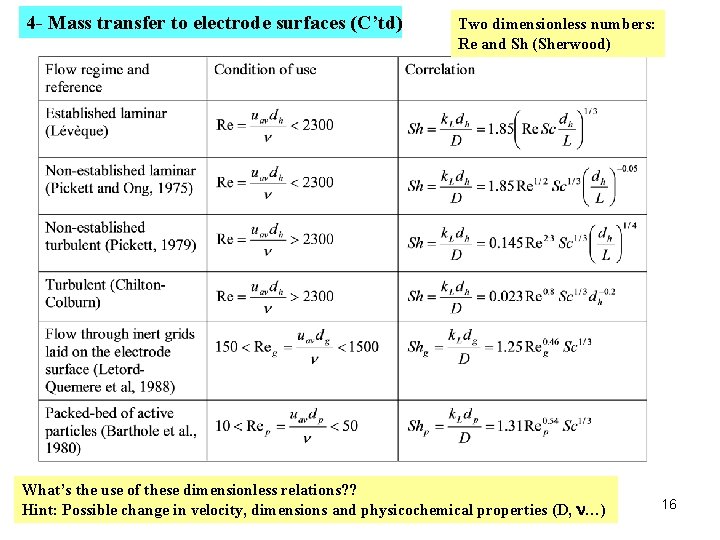
4 - Mass transfer to electrode surfaces (C’td) Two dimensionless numbers: Re and Sh (Sherwood) What’s the use of these dimensionless relations? ? Hint: Possible change in velocity, dimensions and physicochemical properties (D, n…) 16
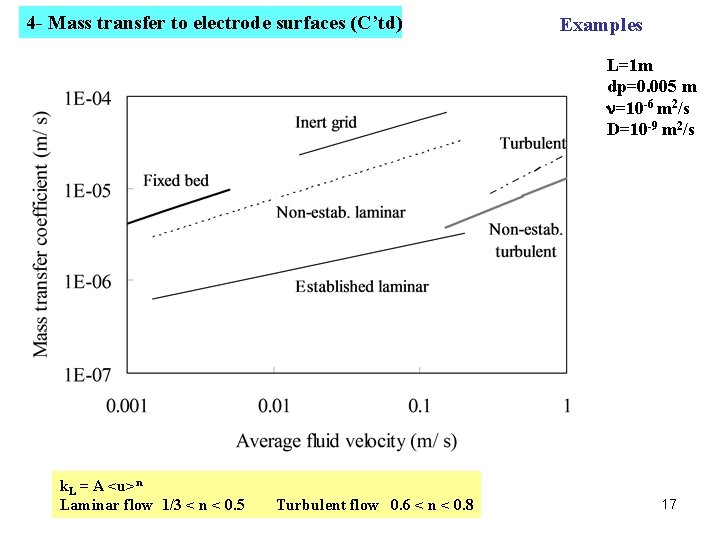
4 - Mass transfer to electrode surfaces (C’td) Examples L=1 m dp=0. 005 m n=10 -6 m 2/s D=10 -9 m 2/s k. L = A <u>n Laminar flow 1/3 < n < 0. 5 Turbulent flow 0. 6 < n < 0. 8 17
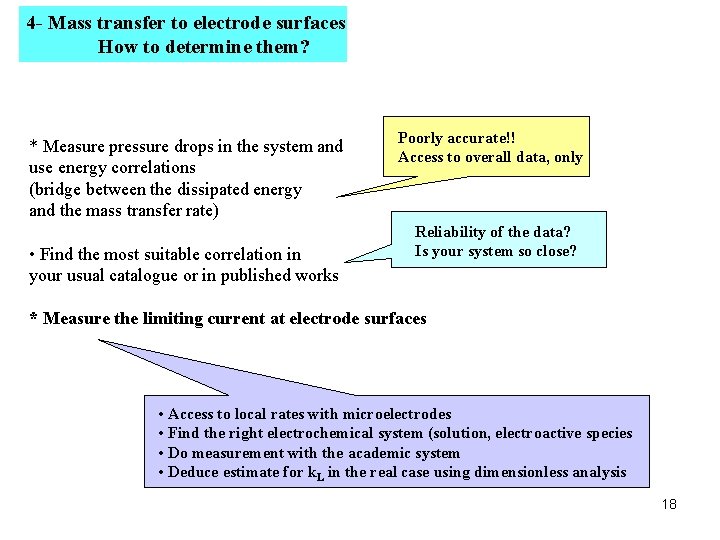
4 - Mass transfer to electrode surfaces How to determine them? * Measure pressure drops in the system and use energy correlations (bridge between the dissipated energy and the mass transfer rate) • Find the most suitable correlation in your usual catalogue or in published works Poorly accurate!! Access to overall data, only Reliability of the data? Is your system so close? * Measure the limiting current at electrode surfaces • Access to local rates with microelectrodes • Find the right electrochemical system (solution, electroactive species • Do measurement with the academic system • Deduce estimate for k. L in the real case using dimensionless analysis 18
Carrier transport phenomena
Slidetodoc.com
Giner electrochemical systems
Cathode vs anode equation
Electrochemical machining advantages and disadvantages
Difference between charge and electric charge
Difference between charge and electric charge
Antiporters
Primary active transport and secondary active transport
Active transport diagram
Electrochemical deposition
Galvanic corrosion
Electrochemical machining animation
Electrochemical gradient
Electrochemical series
Types of electrochemical sensors
The electrochemical series table
Vapor
Difference between dry and wet corrosion