Synchrotron After the cyclotron the next idea was
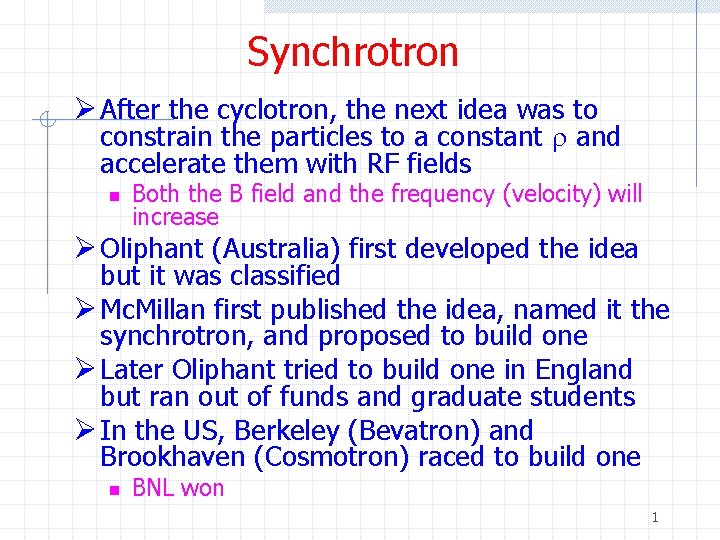
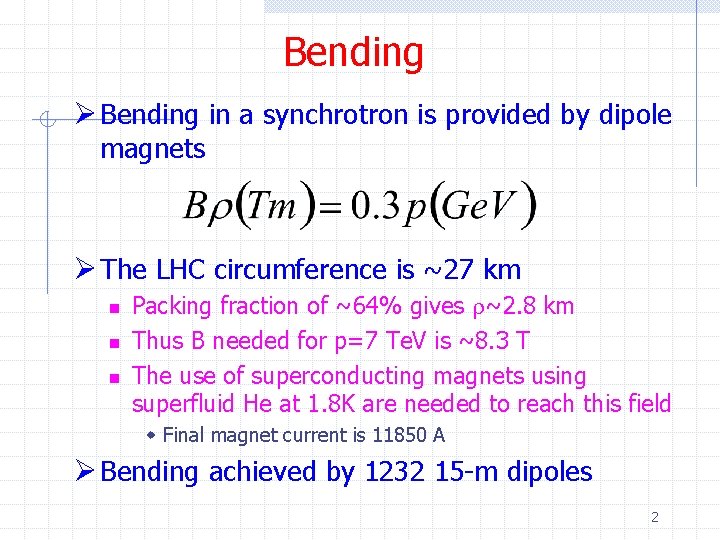
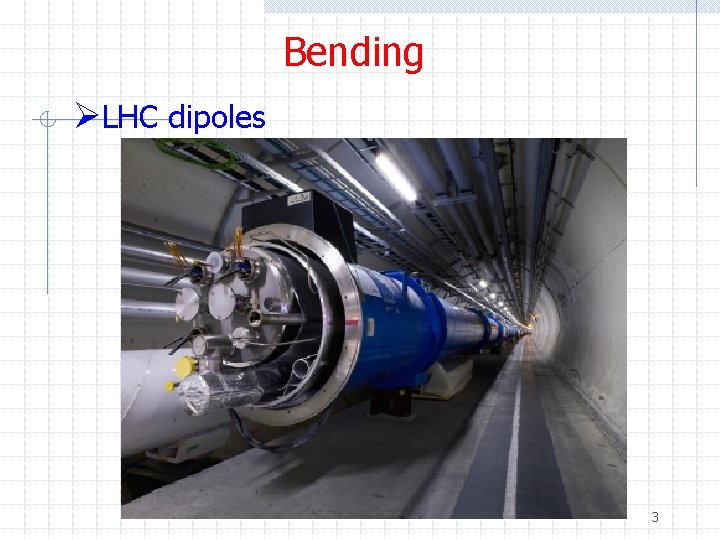
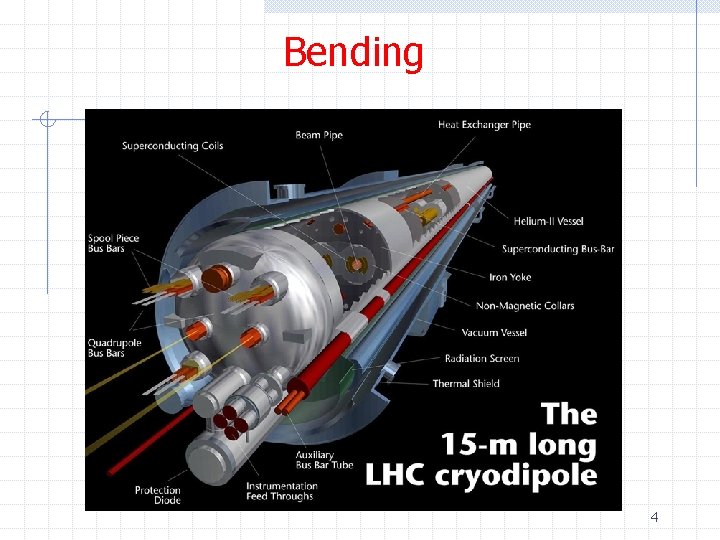
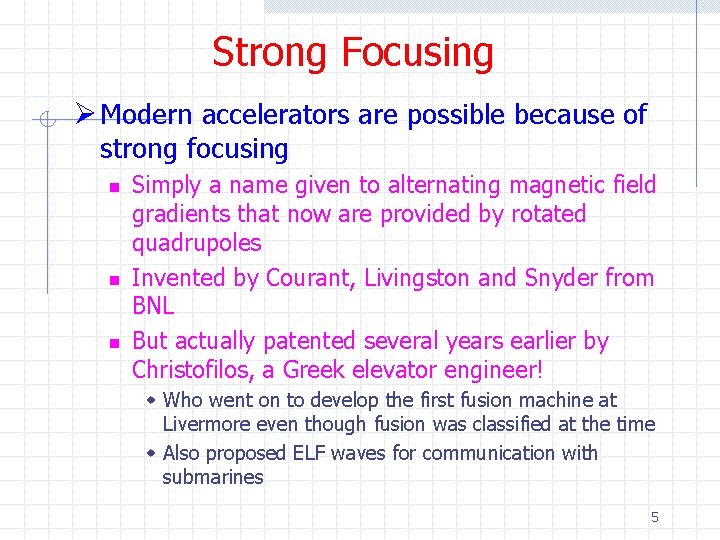
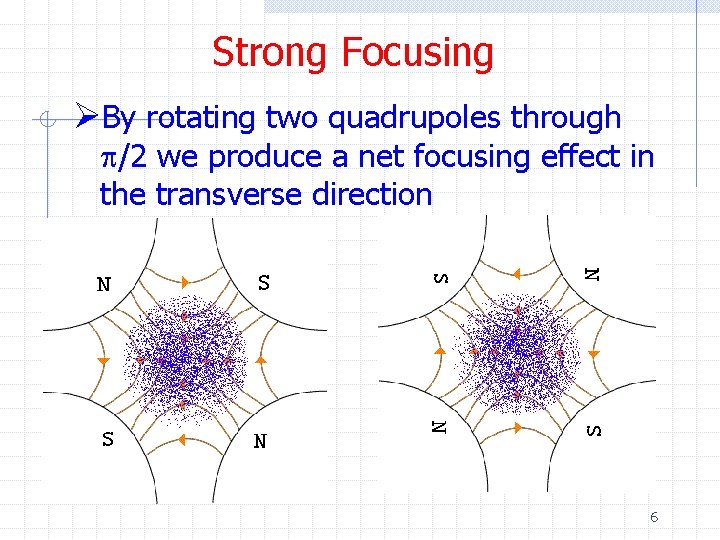
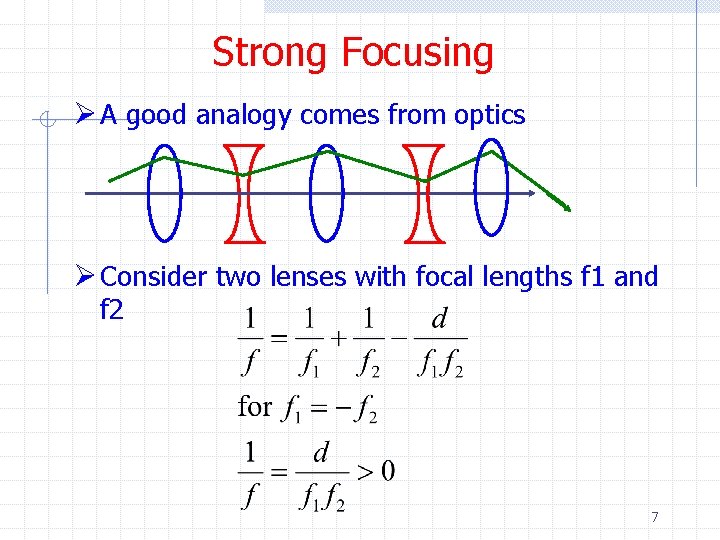
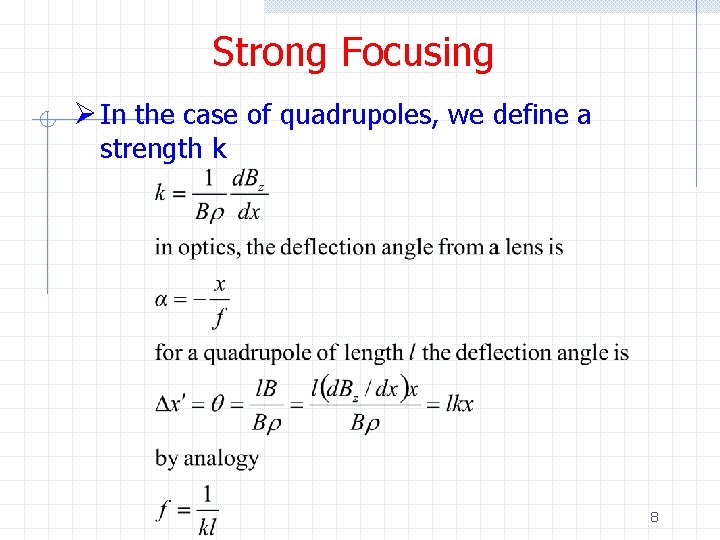
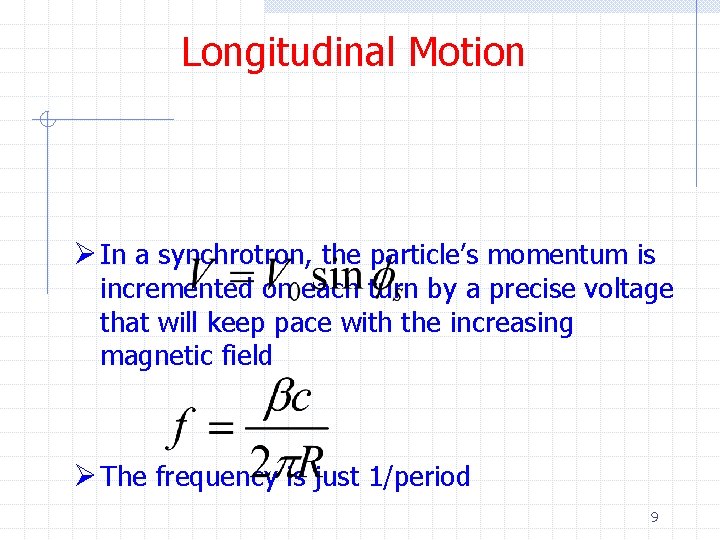
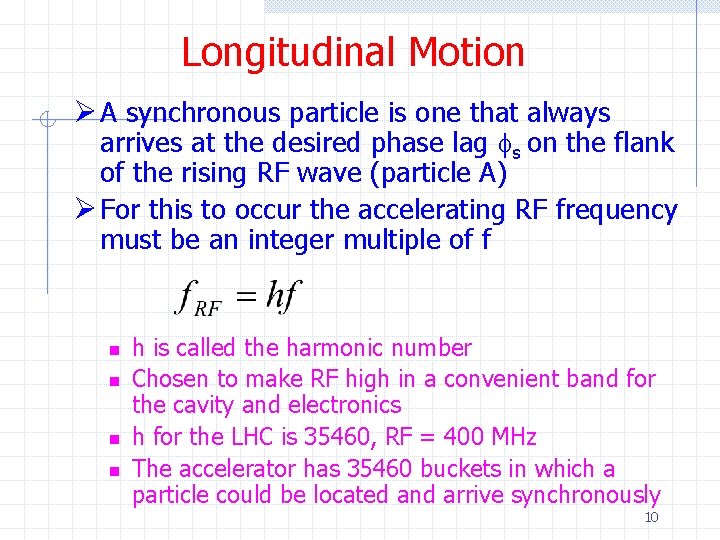
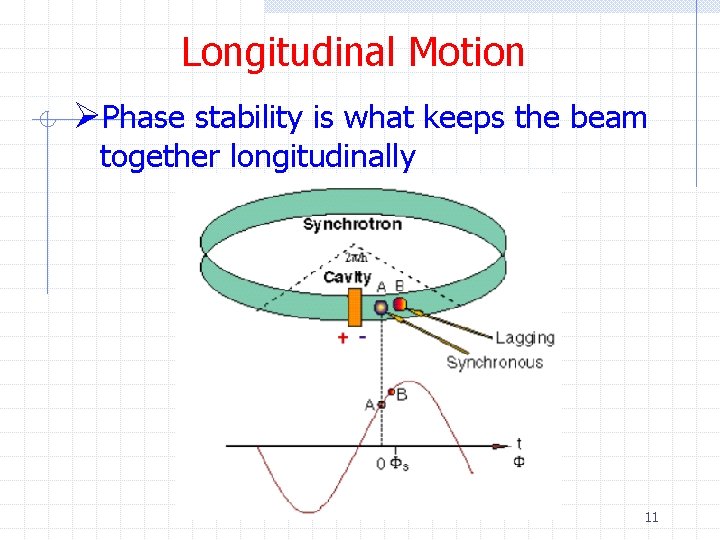
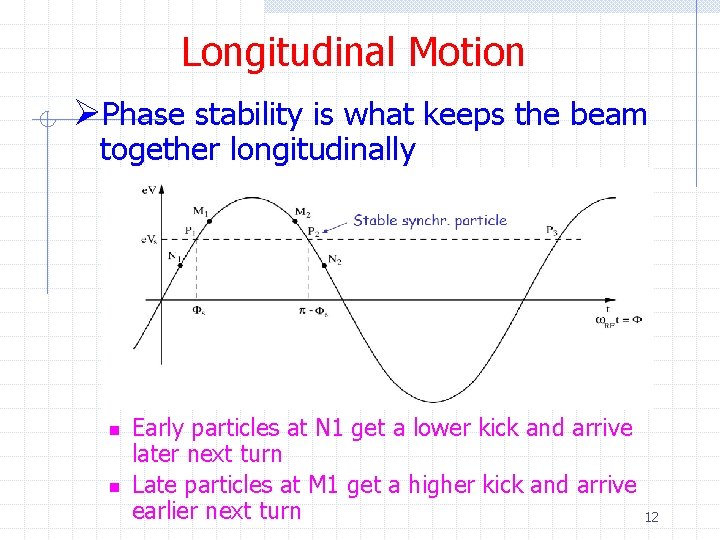
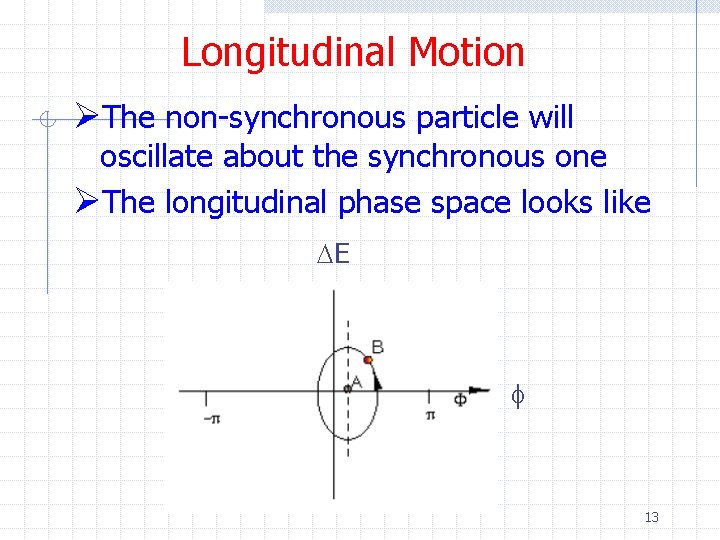
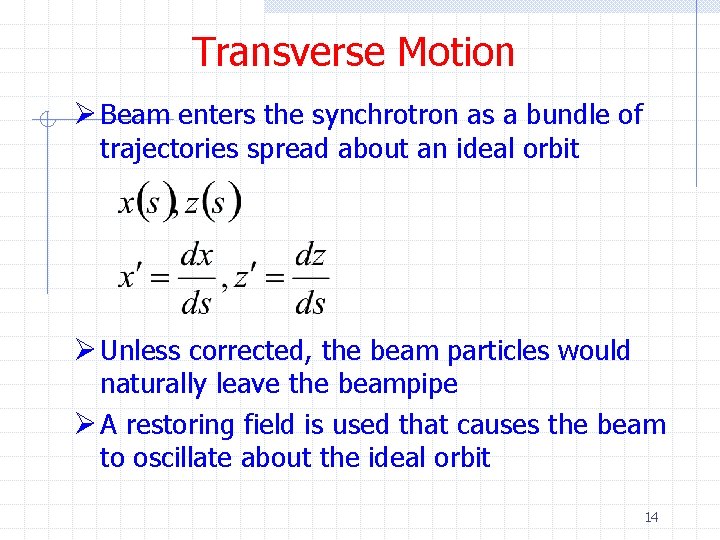
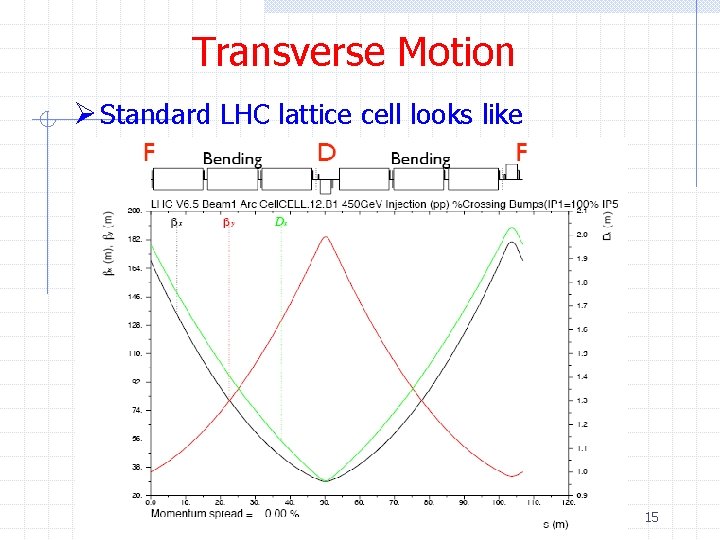
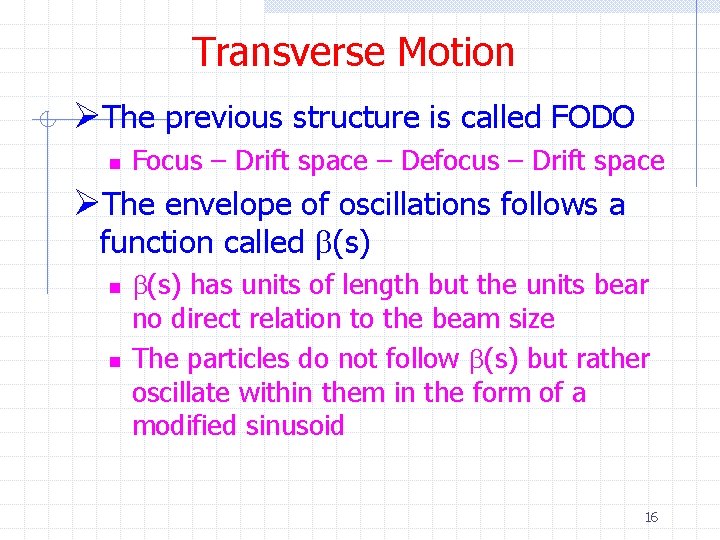
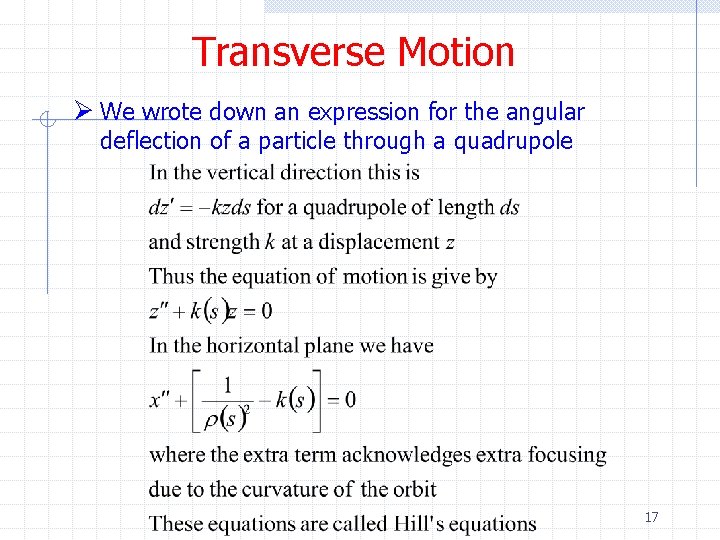
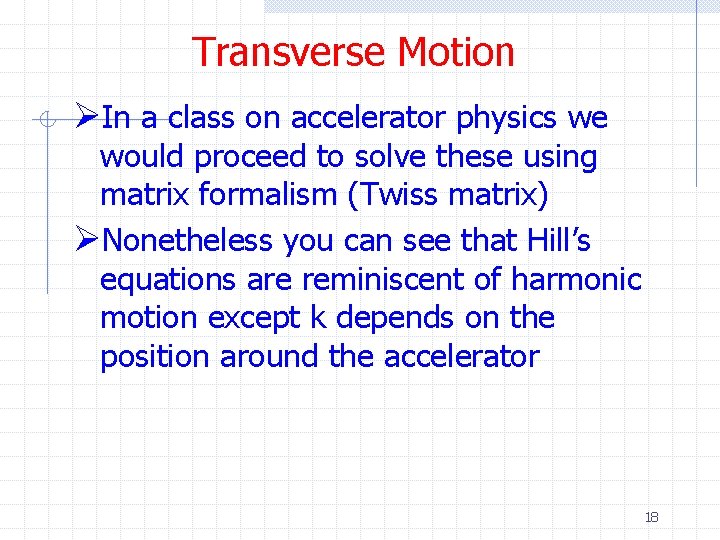
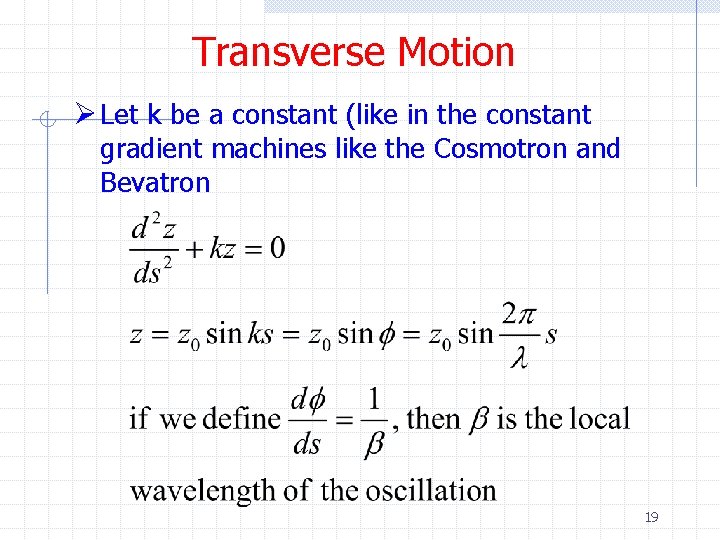
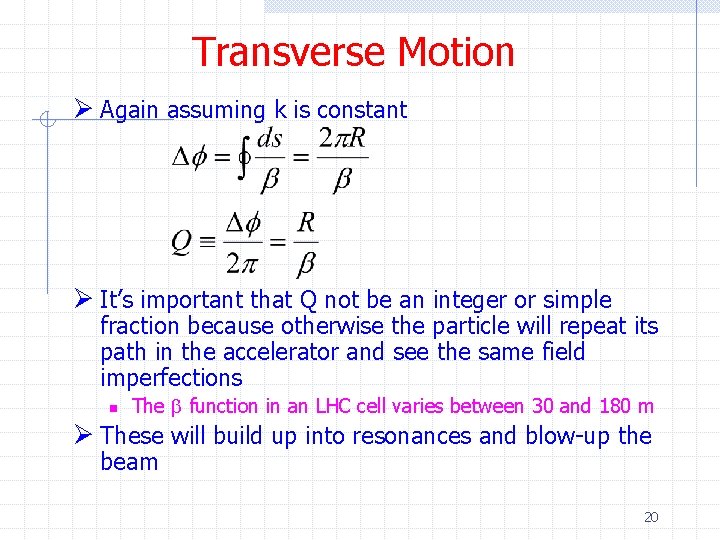
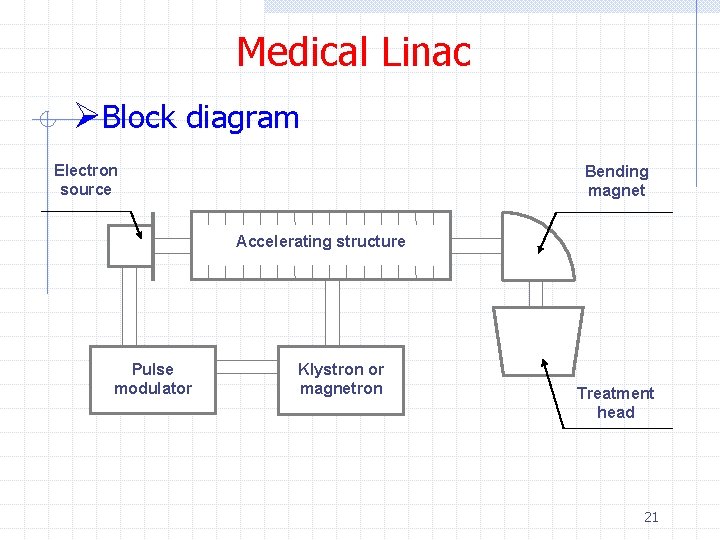
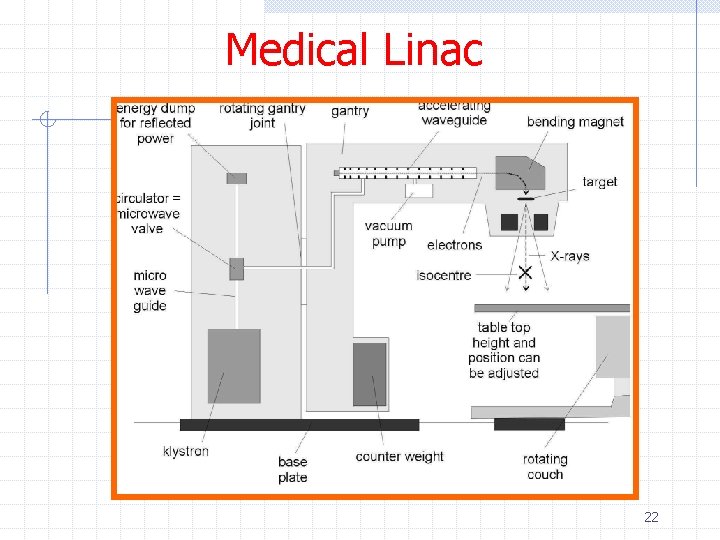
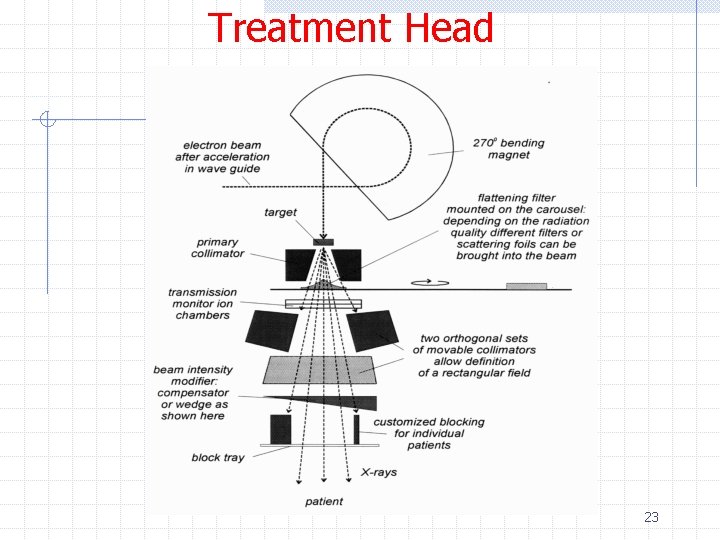
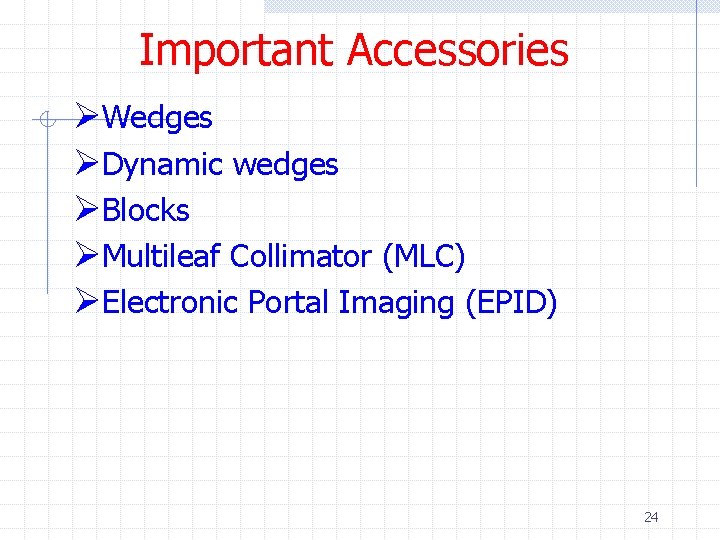
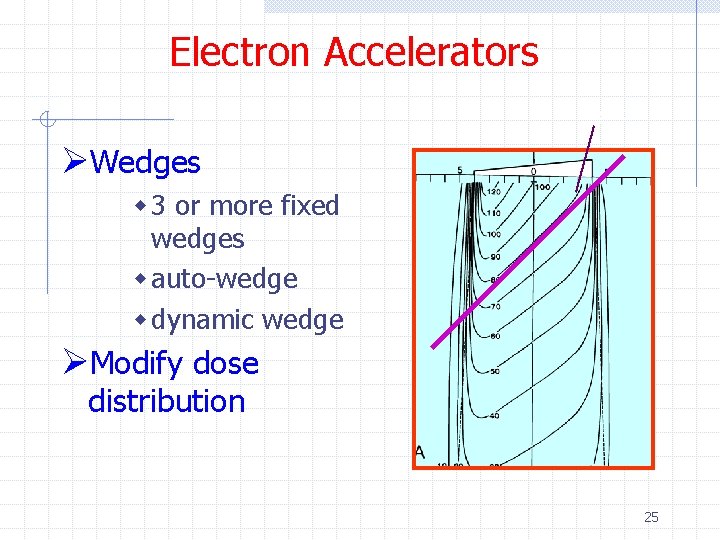
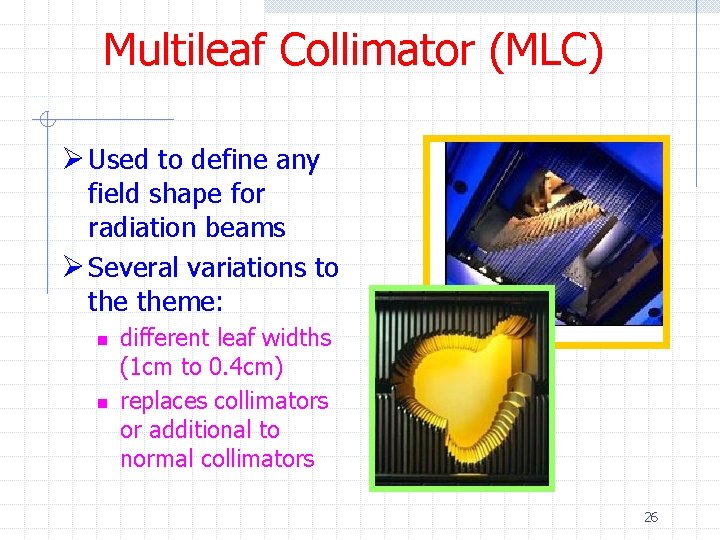
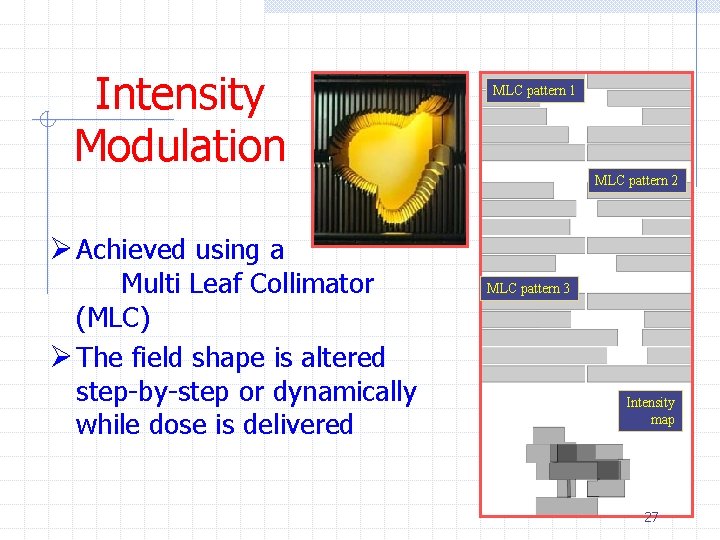
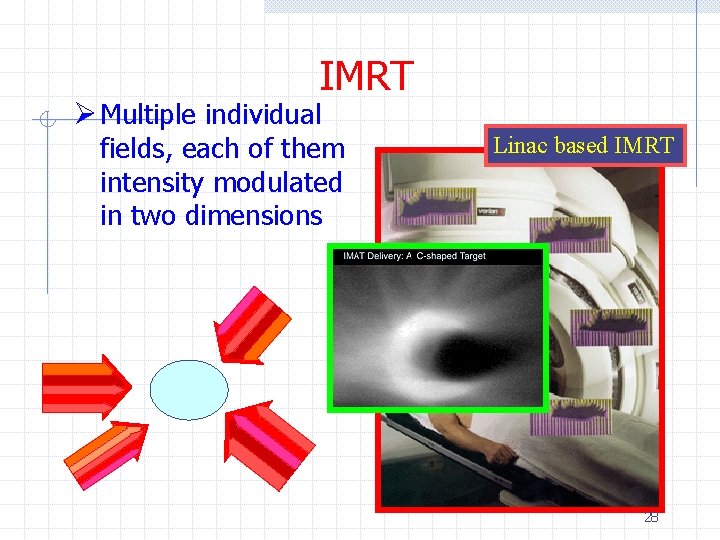
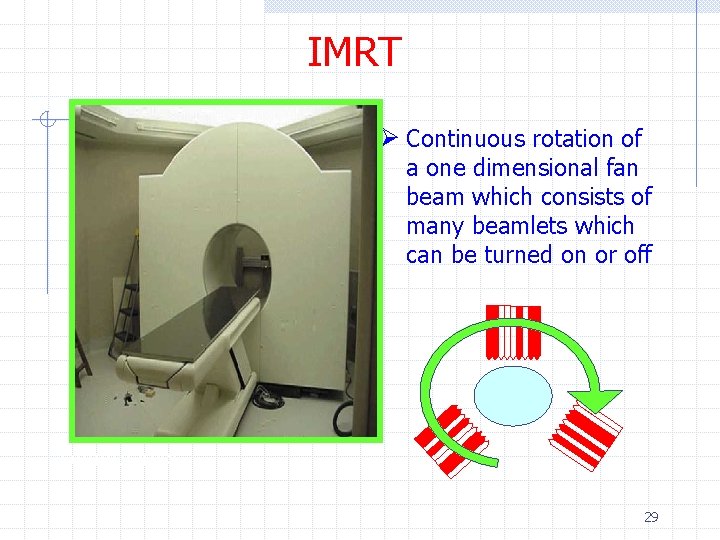
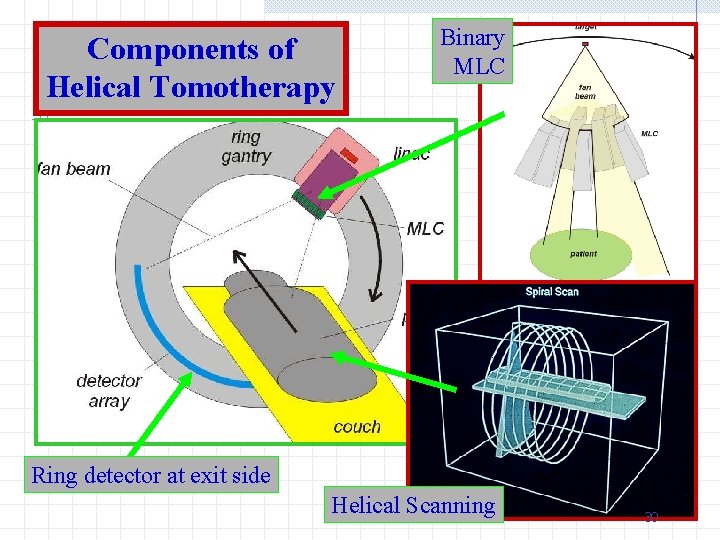
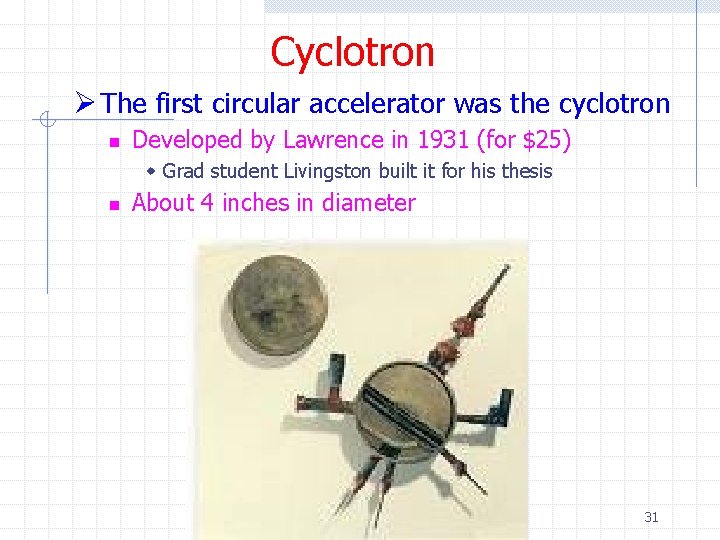
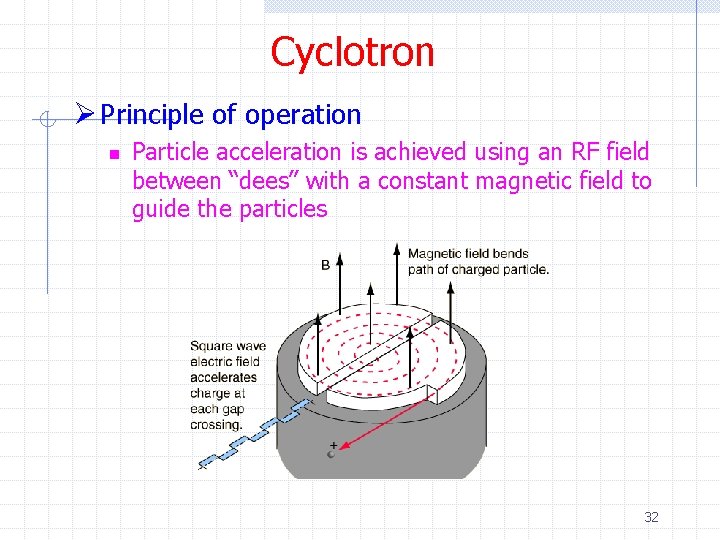
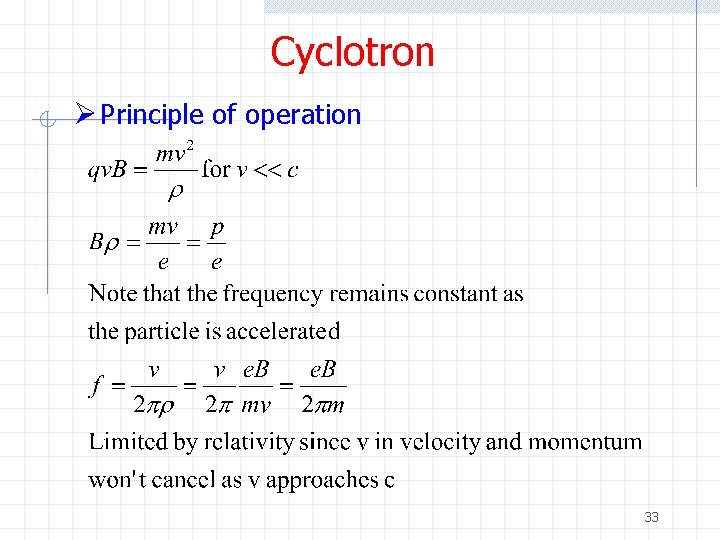
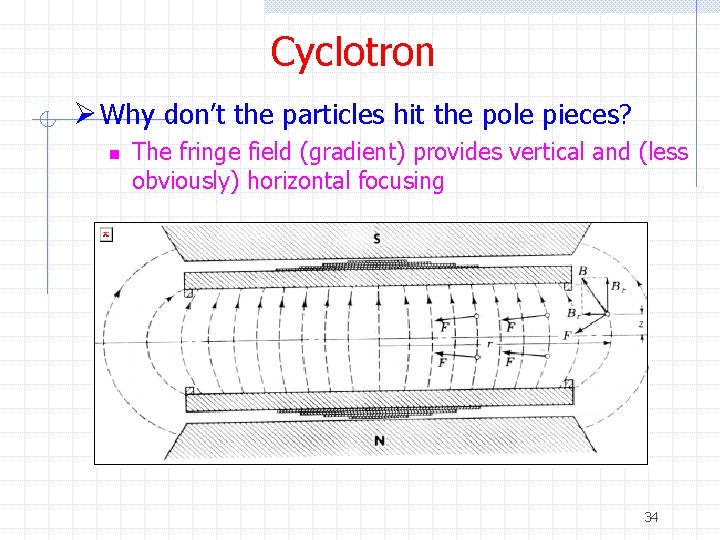
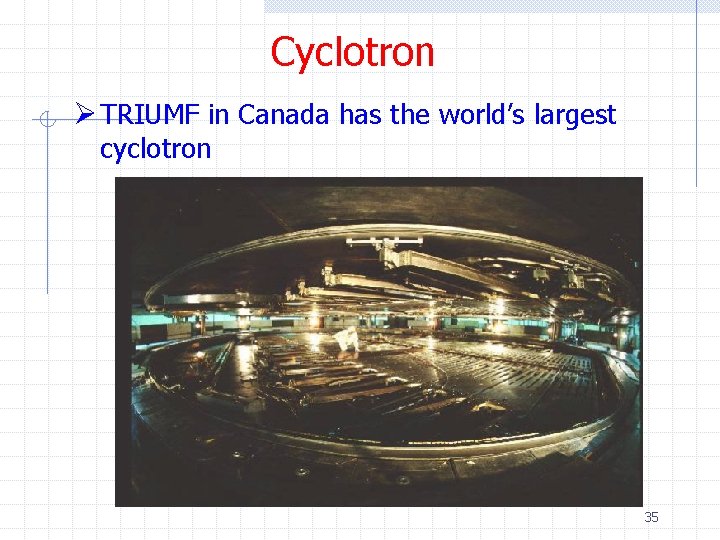
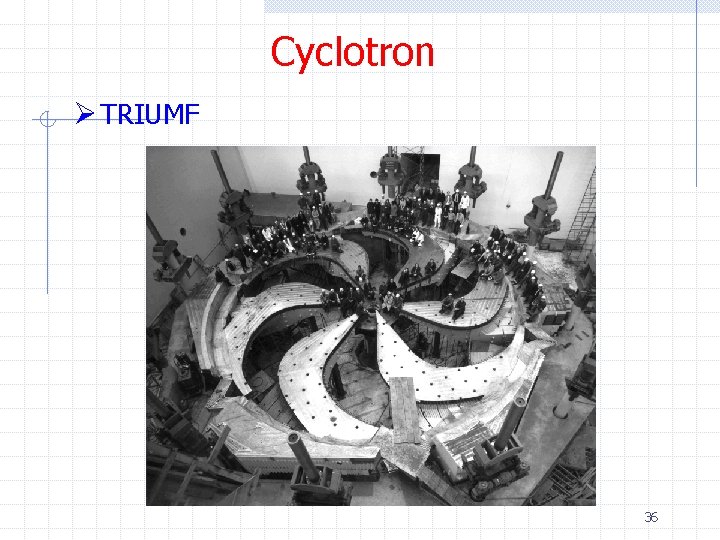
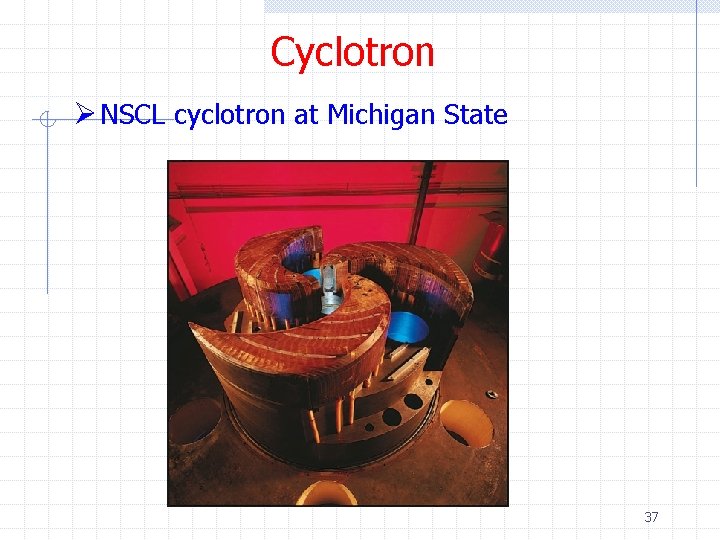
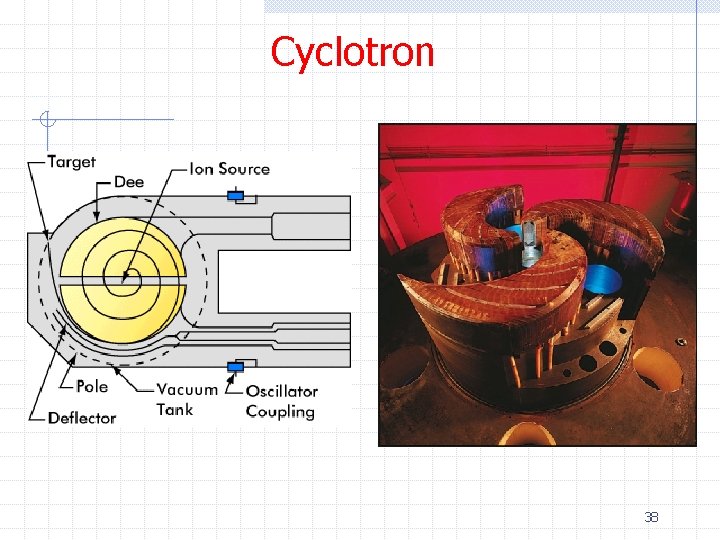
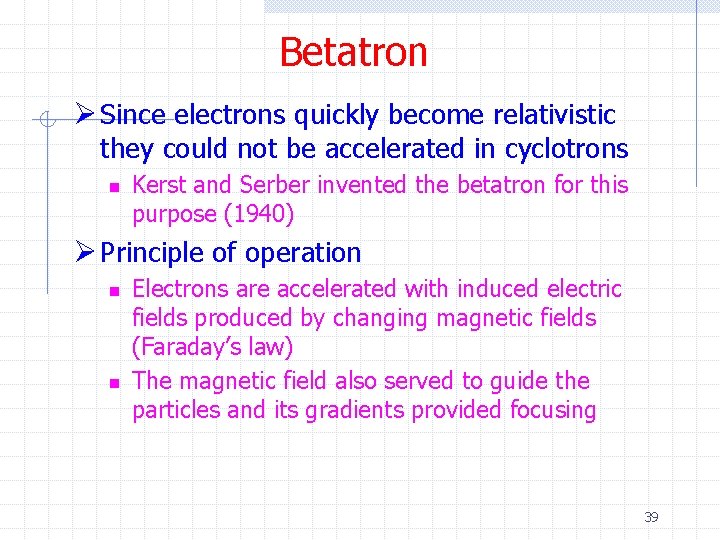
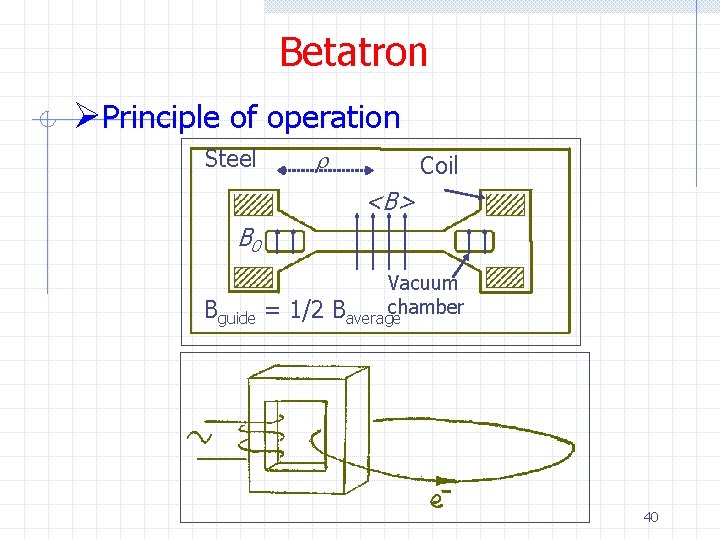
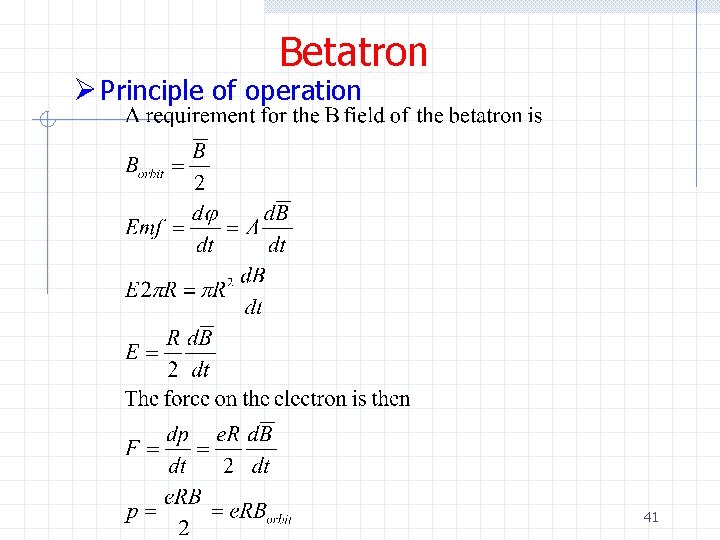
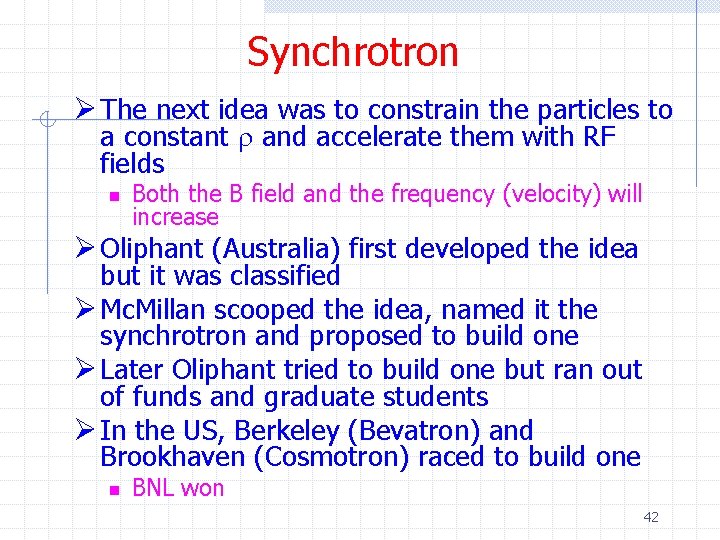
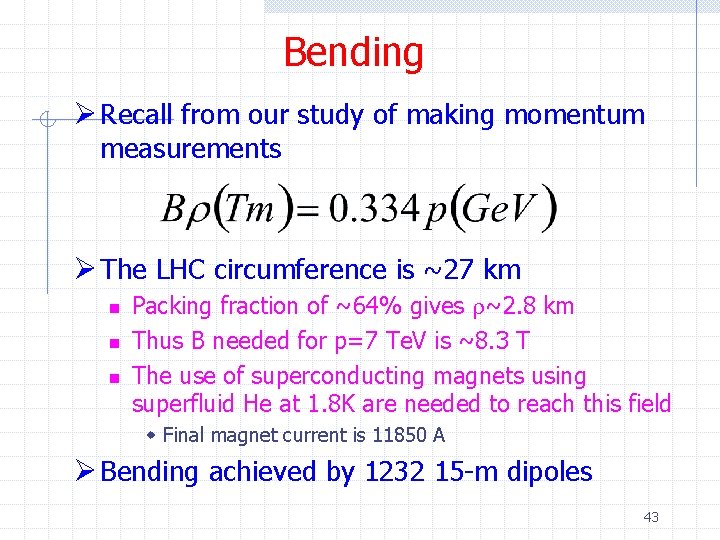
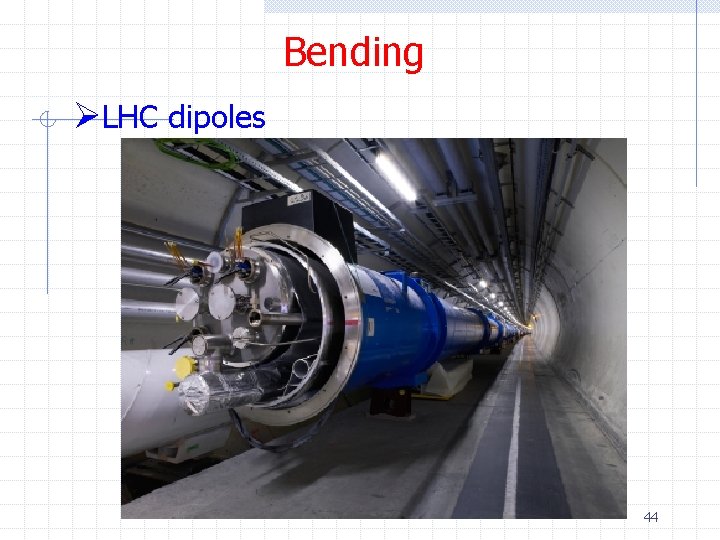
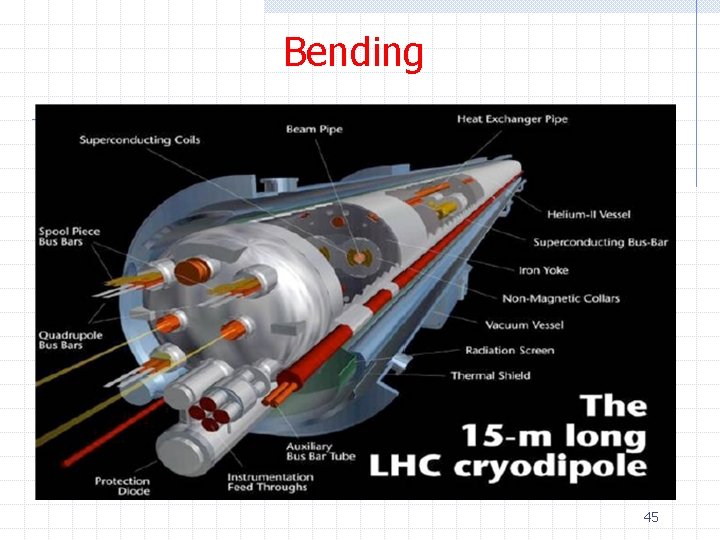
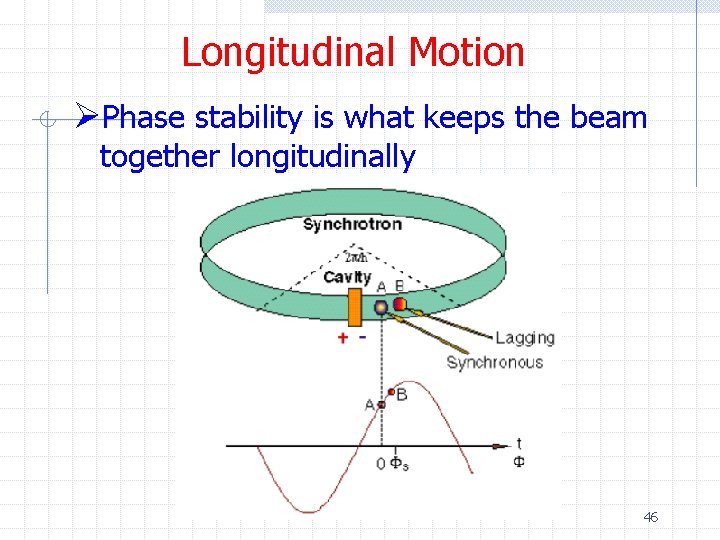
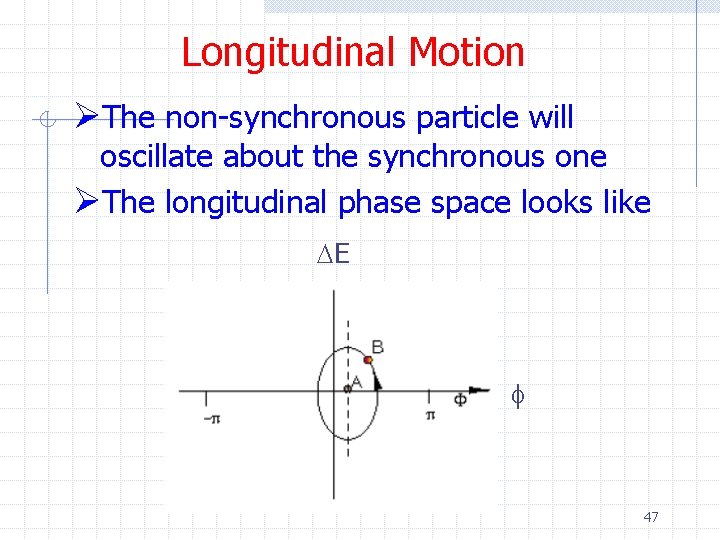
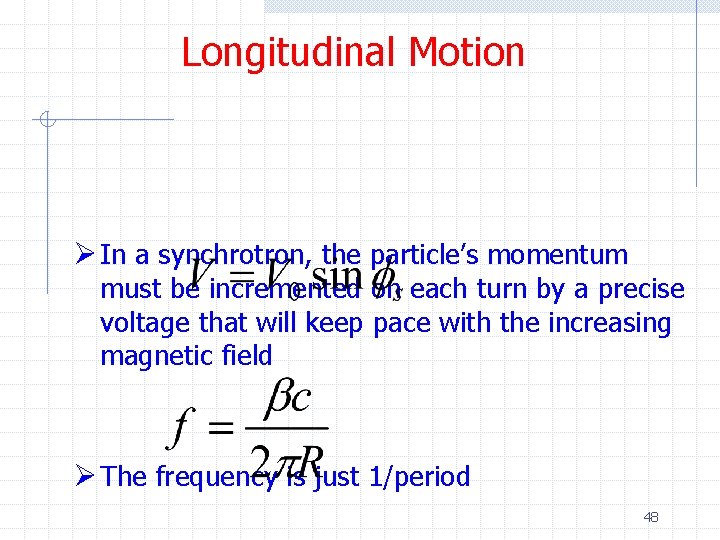
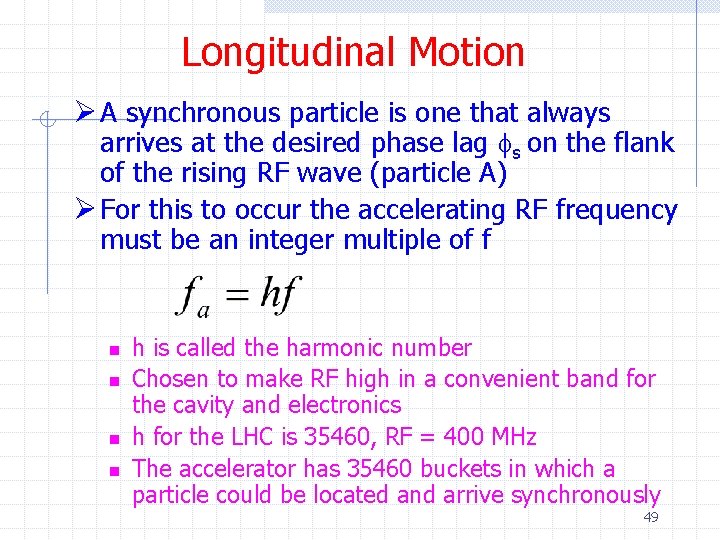
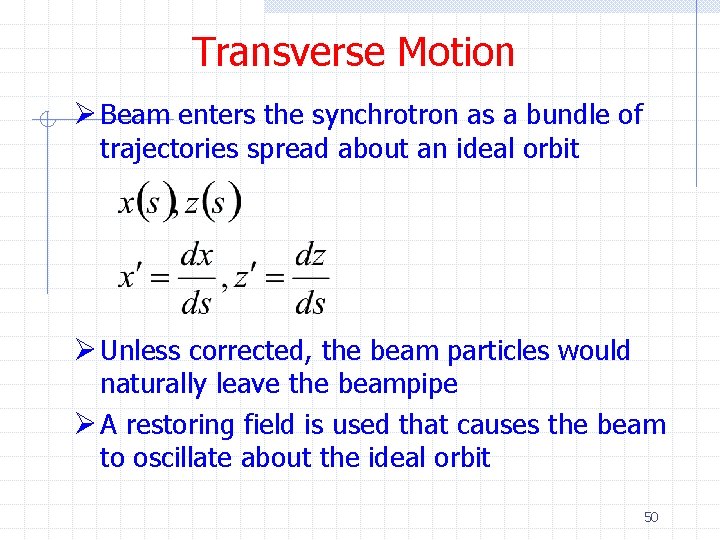
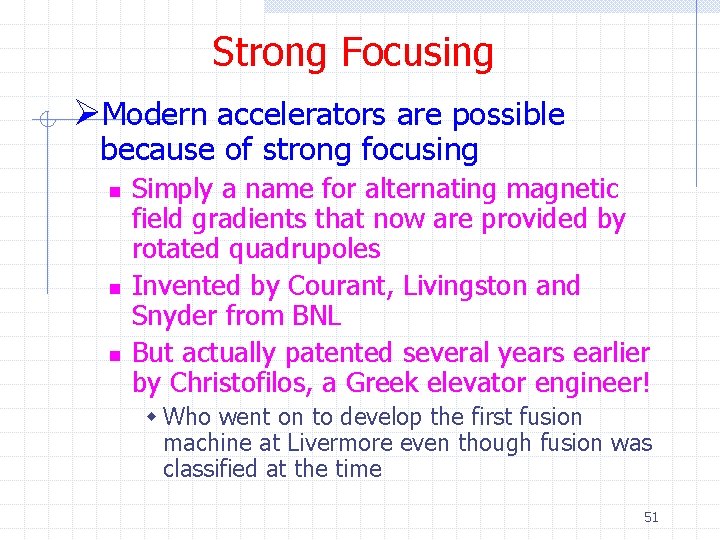
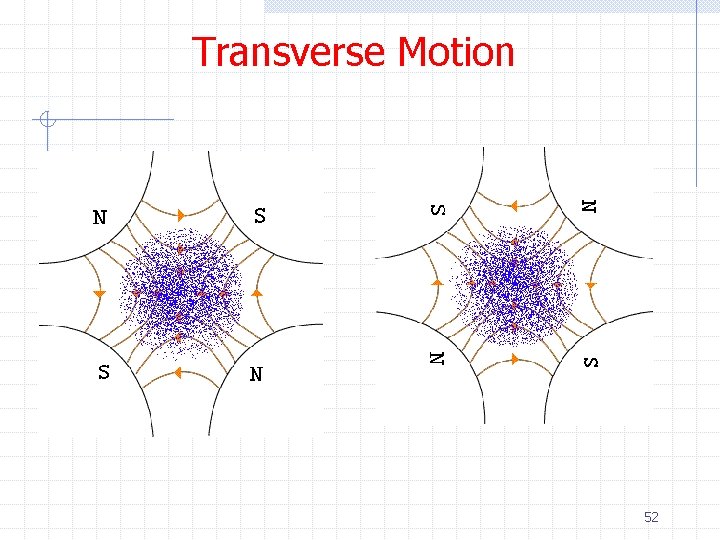
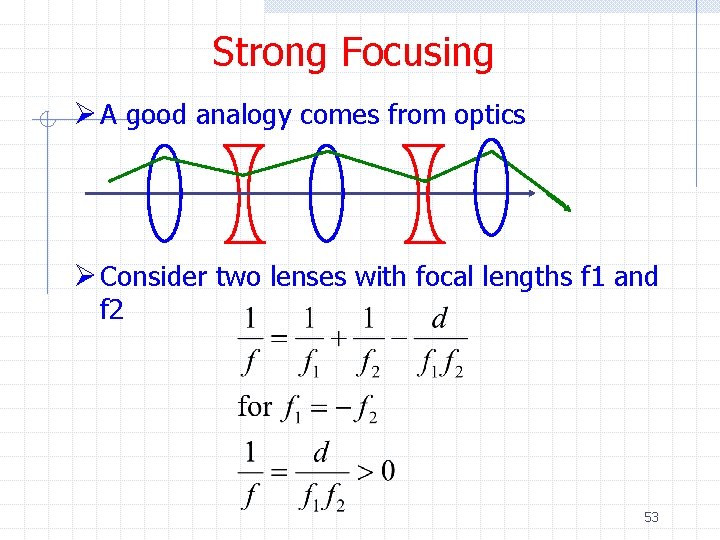
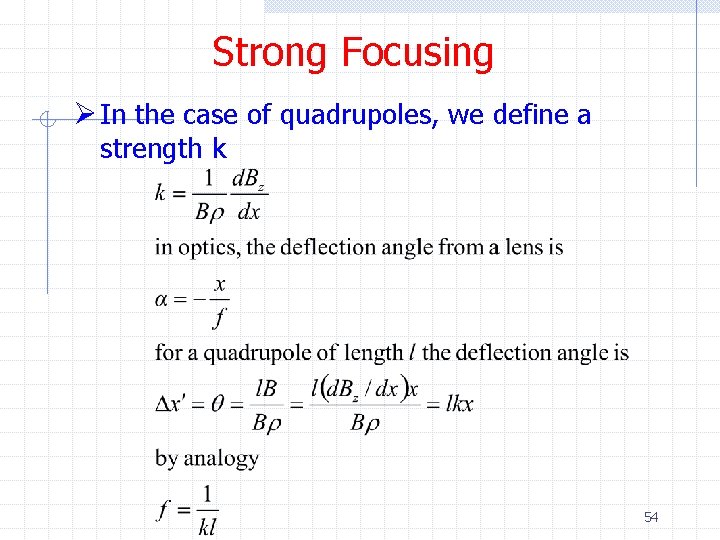
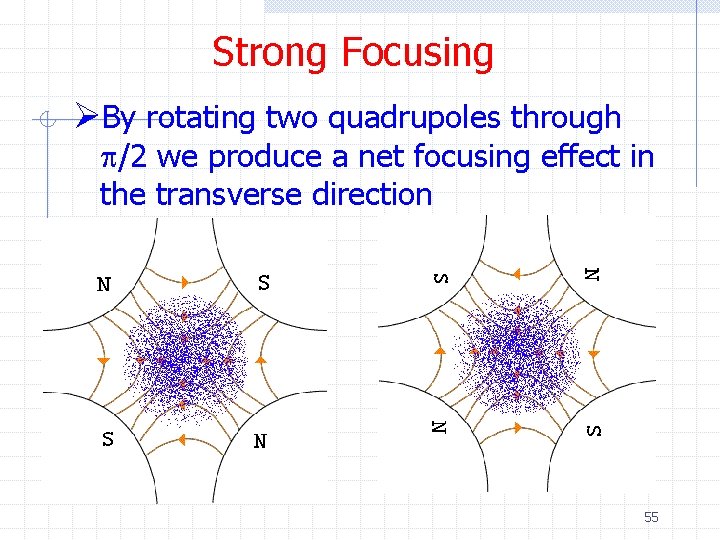
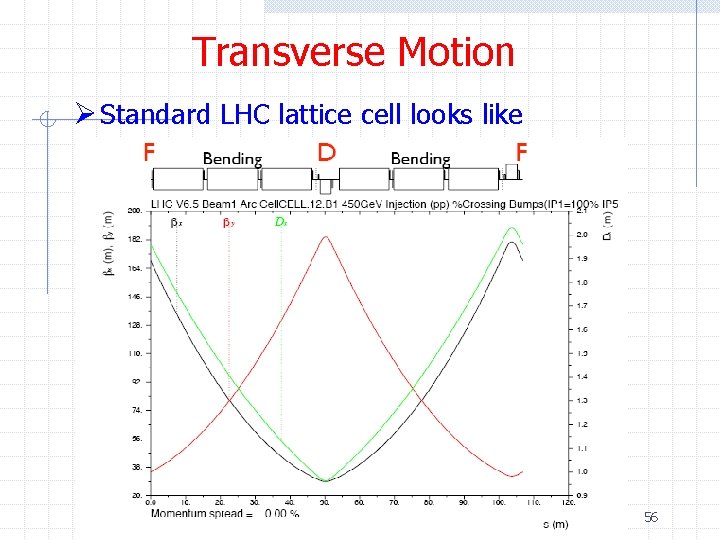
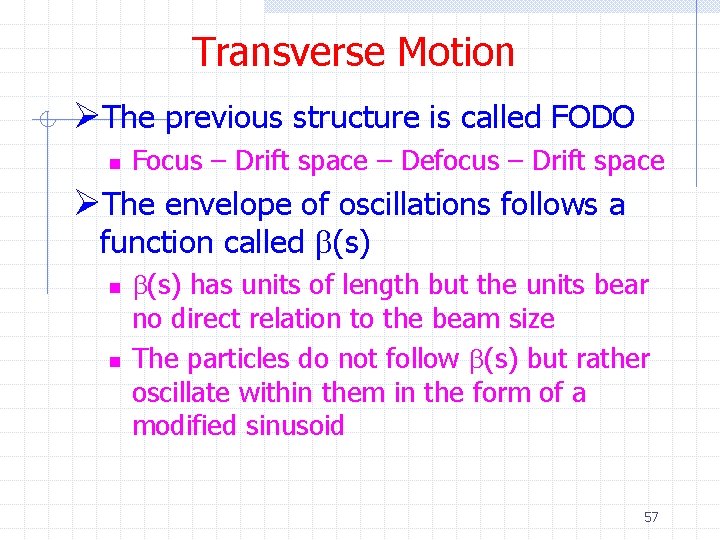
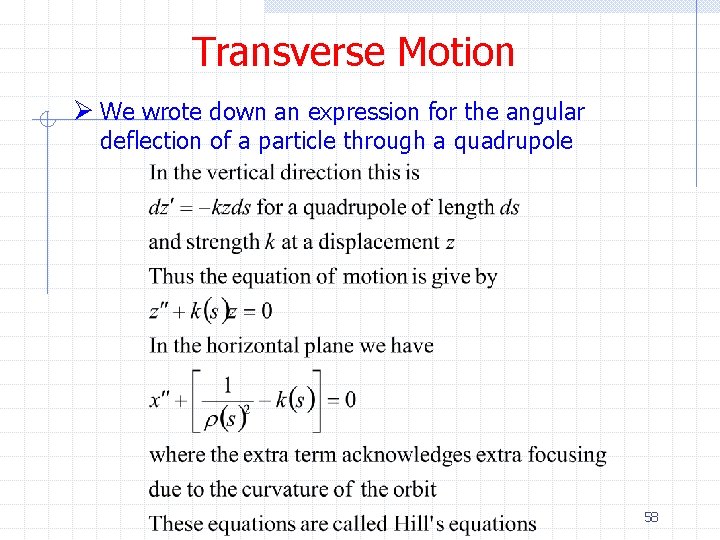
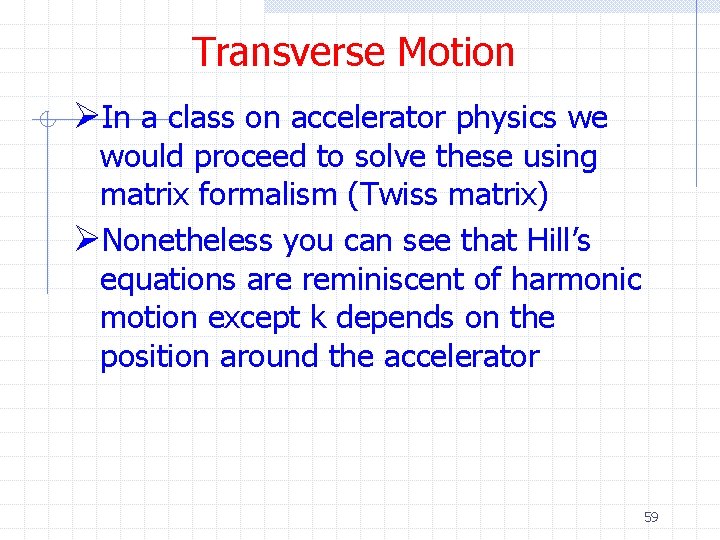
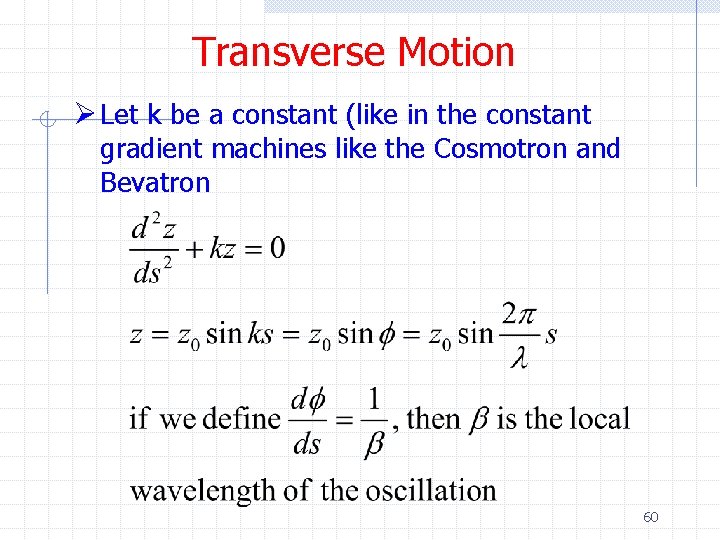
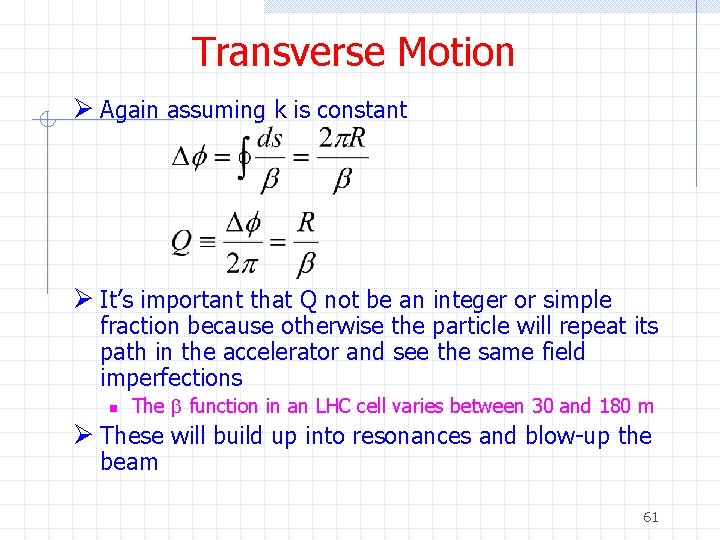
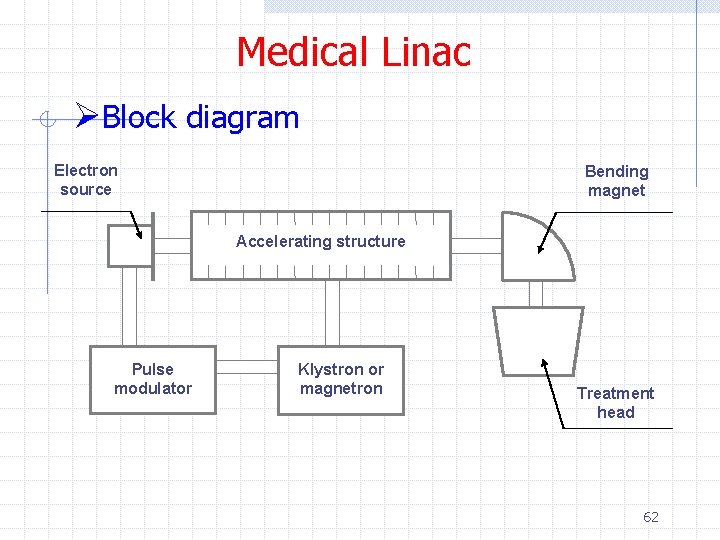
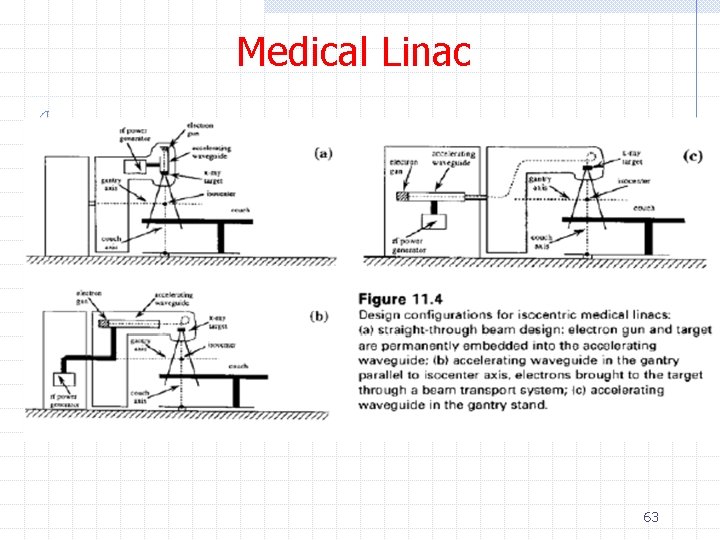
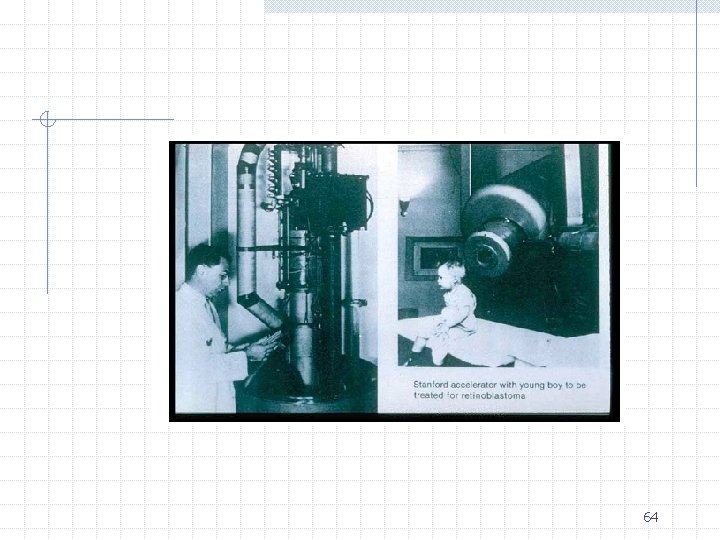
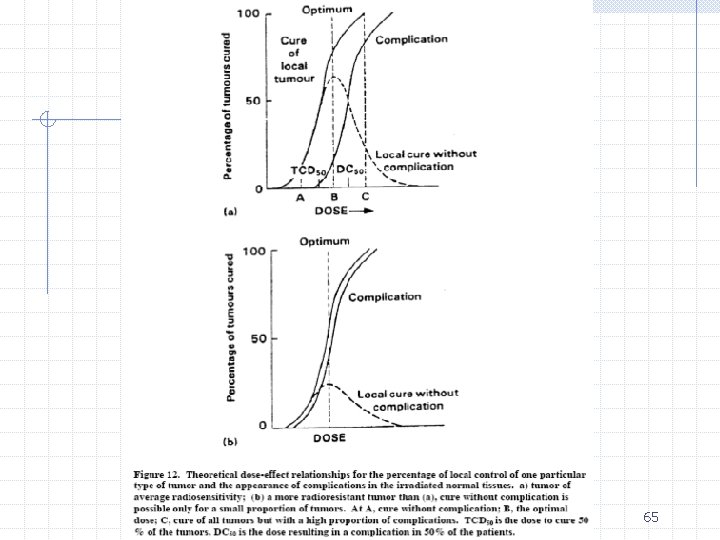
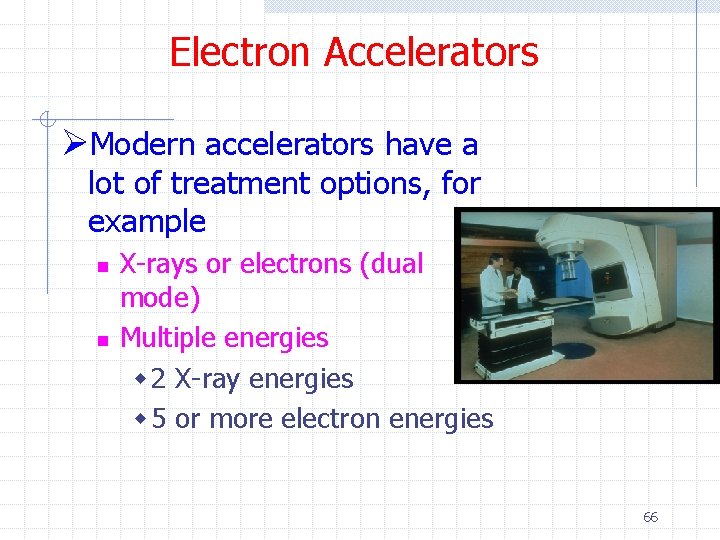
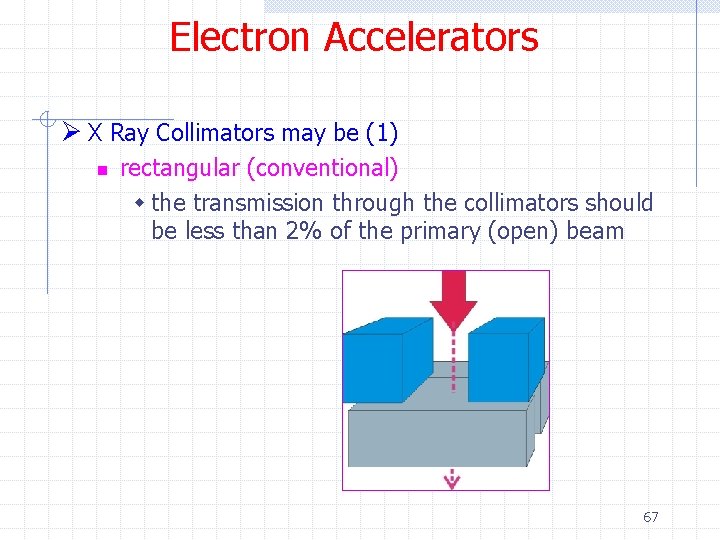
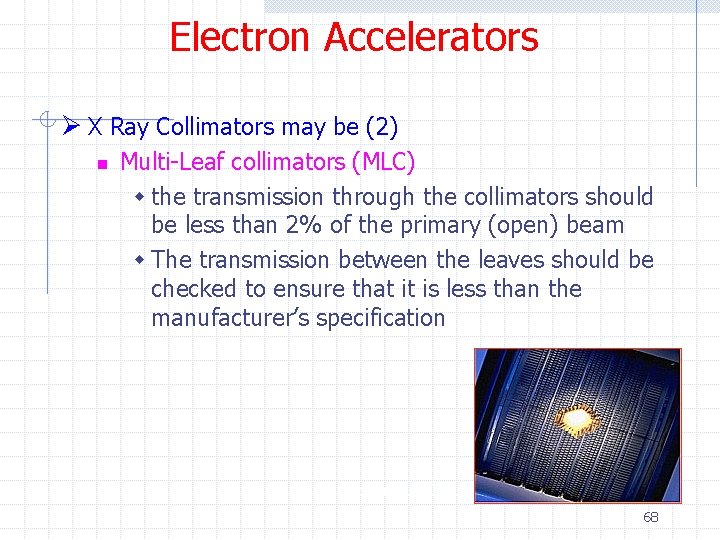
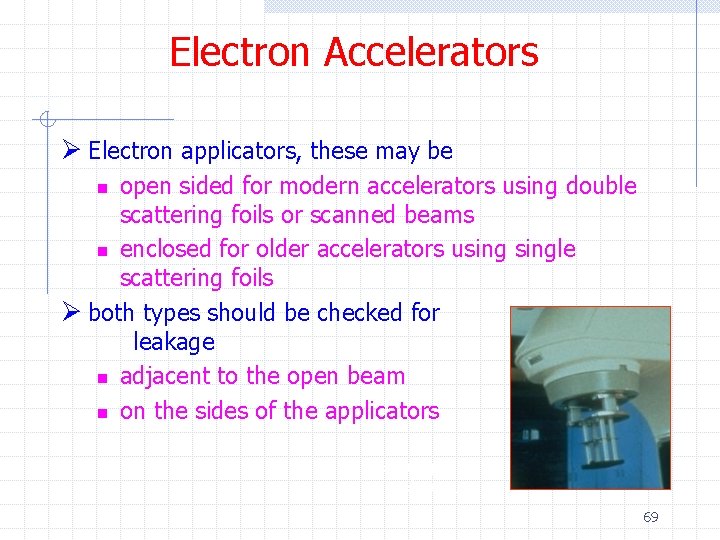
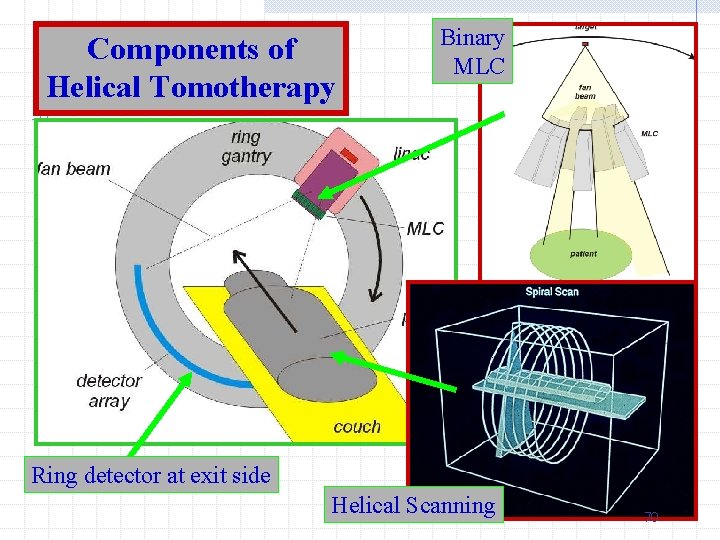
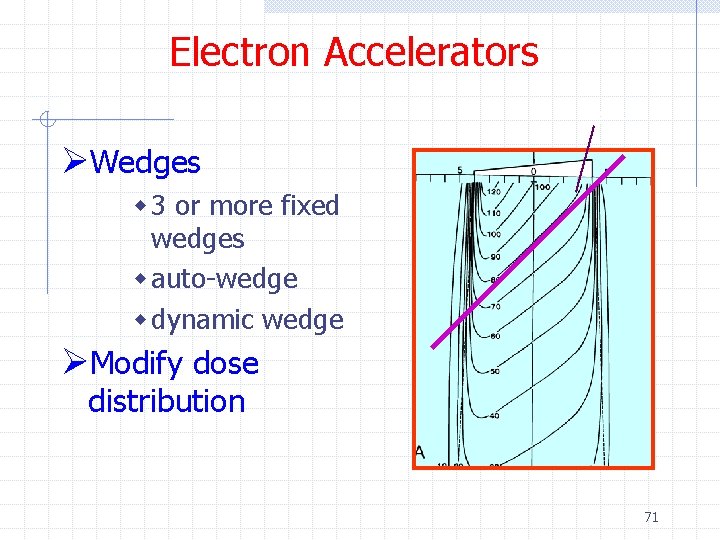
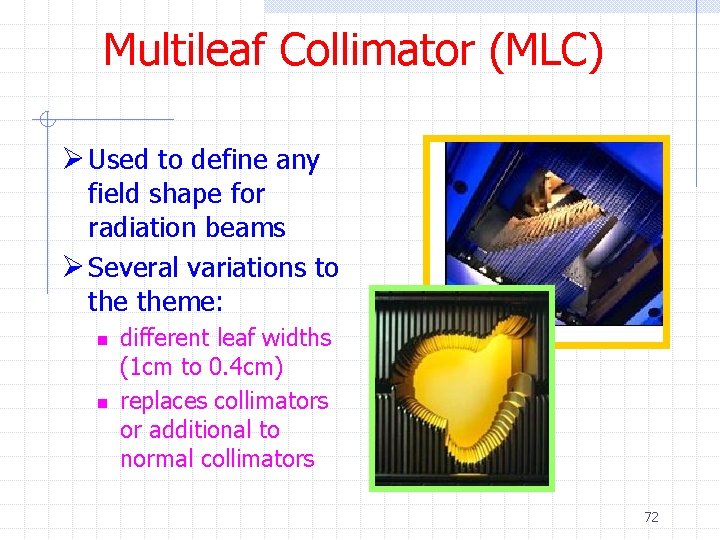
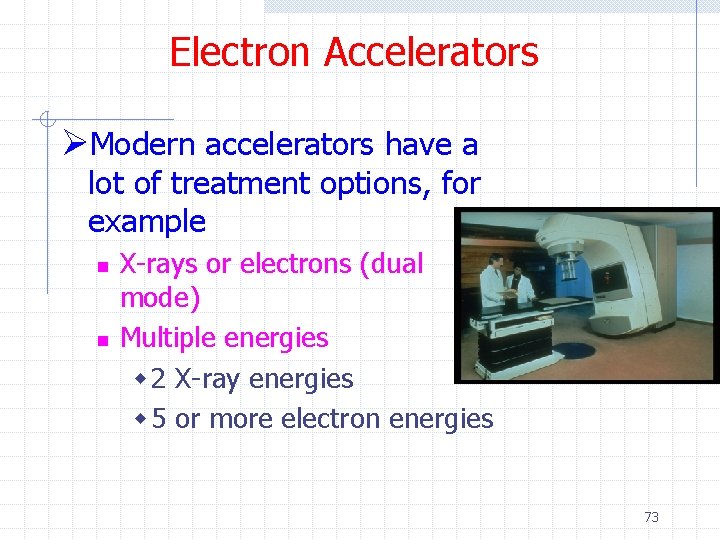
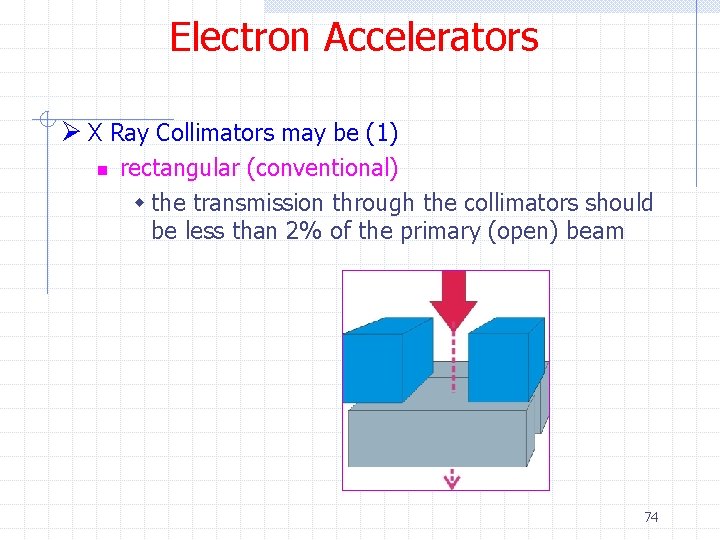
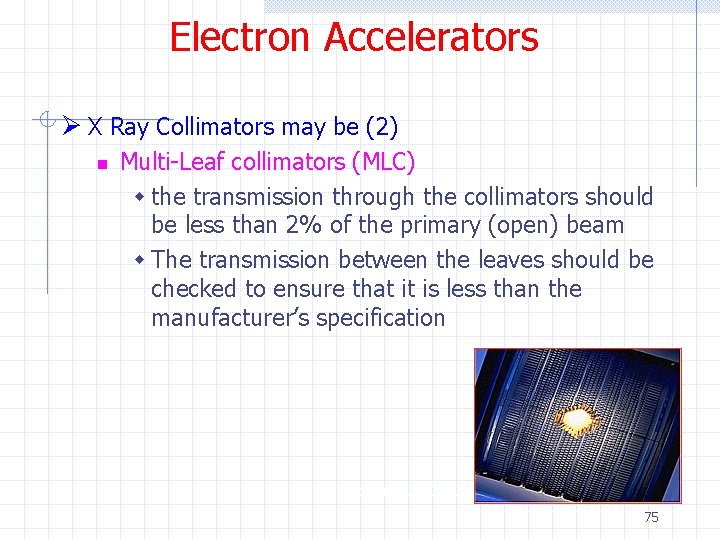
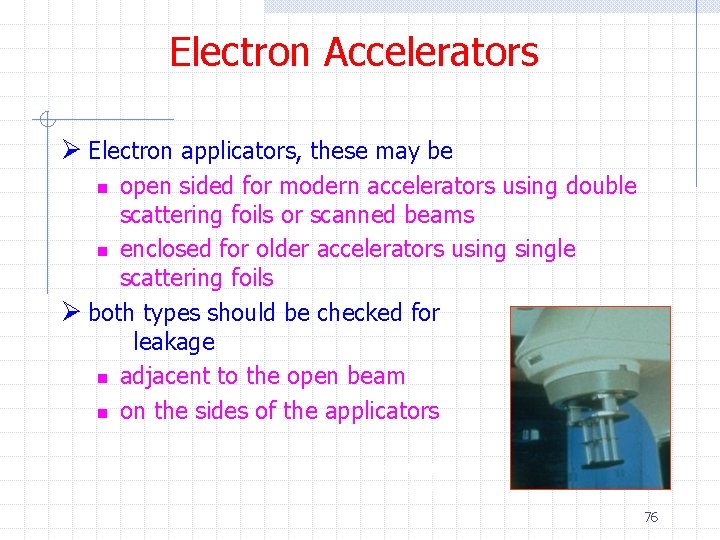
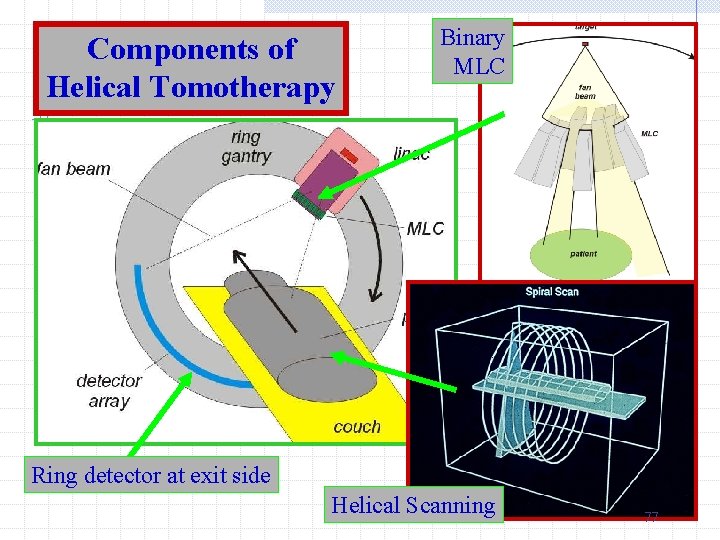
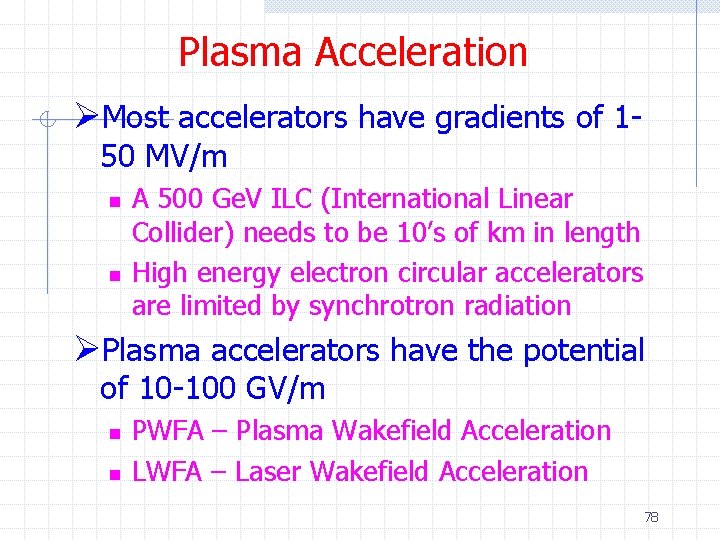
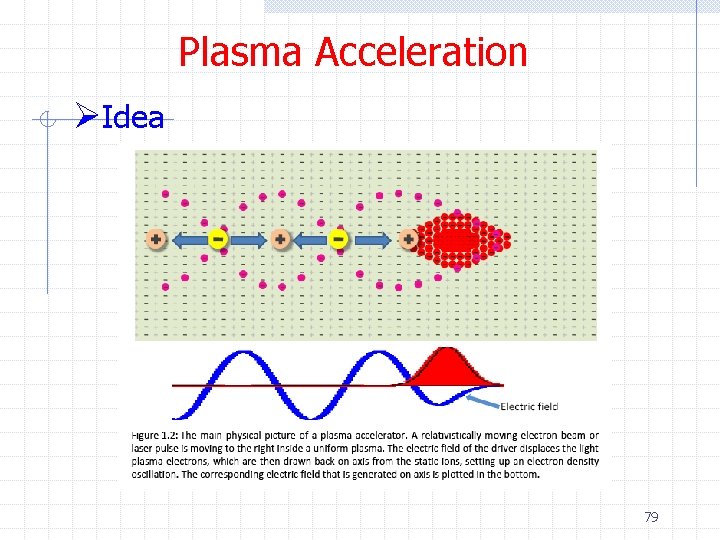
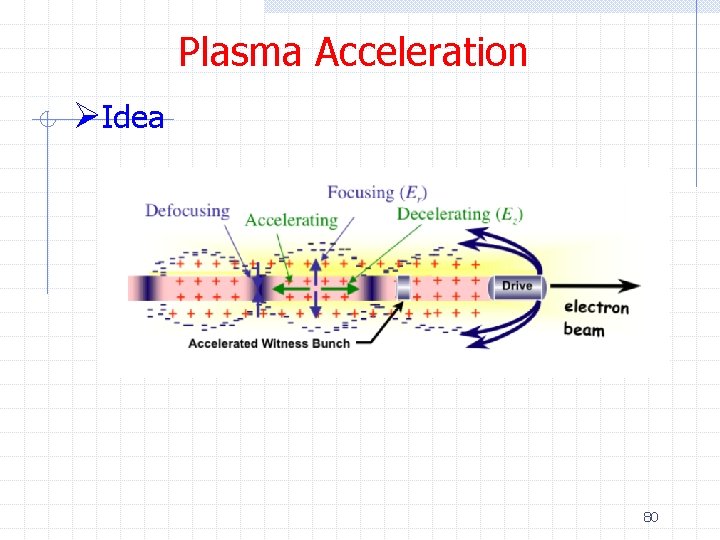
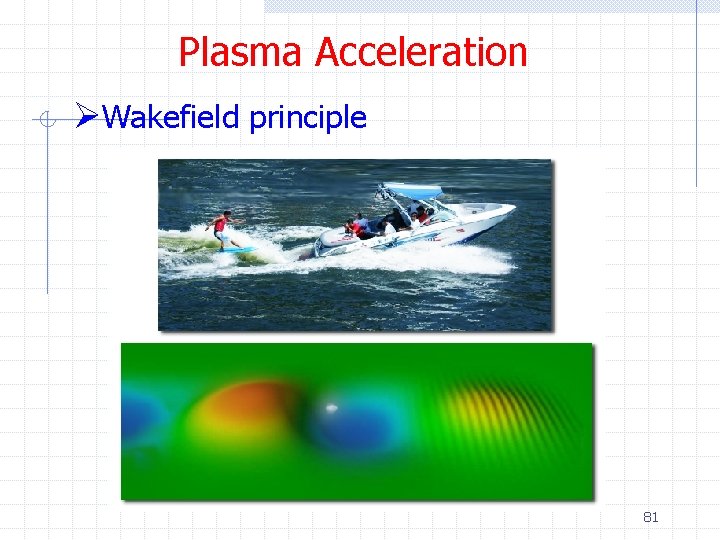
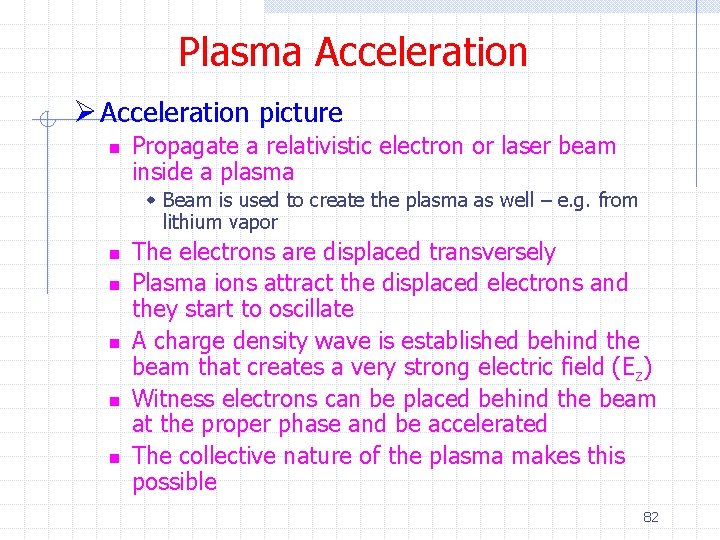
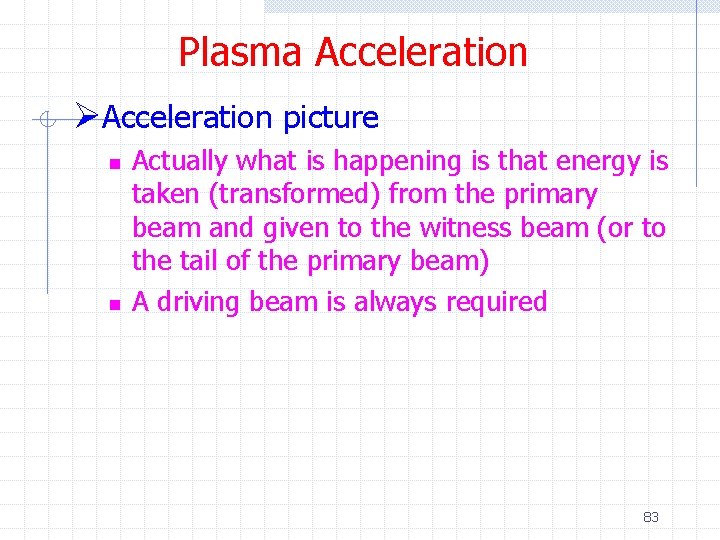
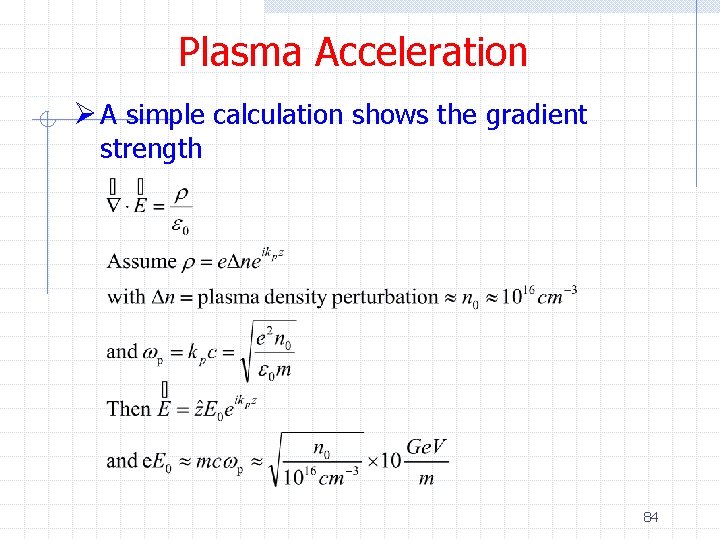
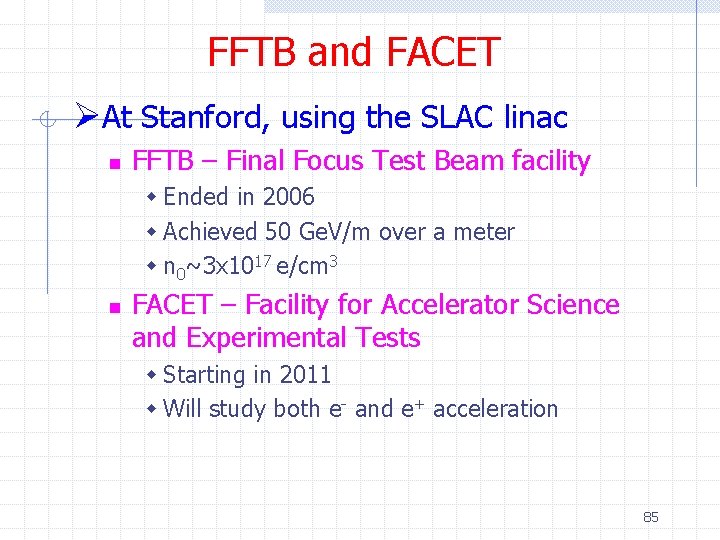
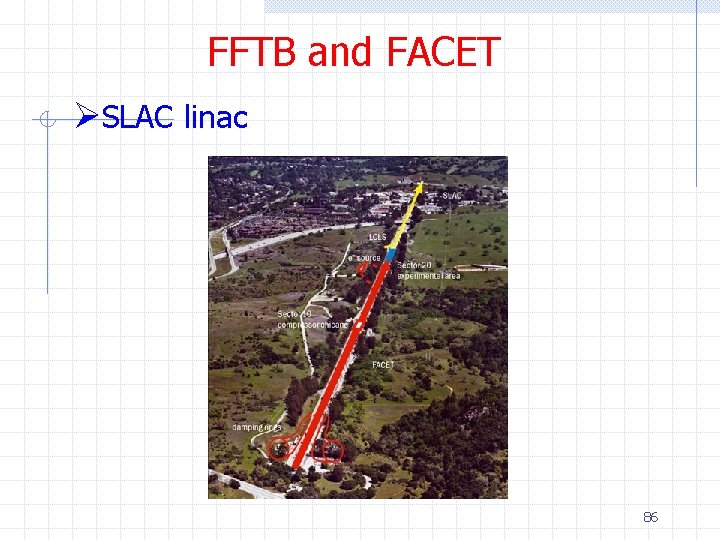
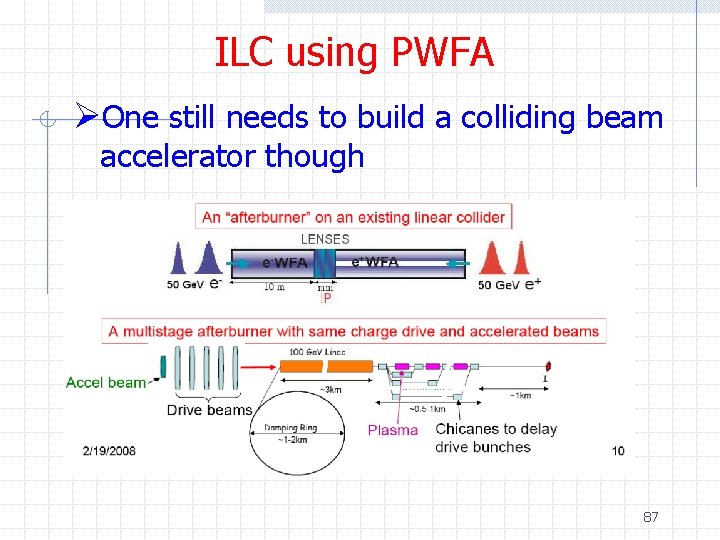
- Slides: 87
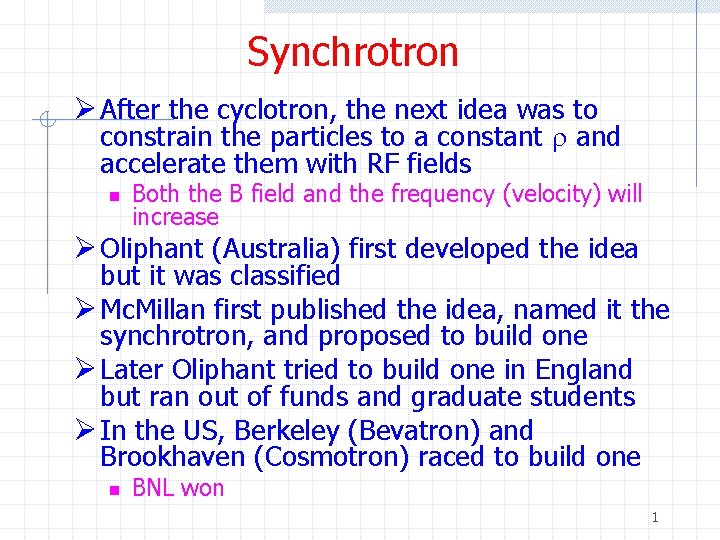
Synchrotron Ø After the cyclotron, the next idea was to constrain the particles to a constant r and accelerate them with RF fields n Both the B field and the frequency (velocity) will increase Ø Oliphant (Australia) first developed the idea but it was classified Ø Mc. Millan first published the idea, named it the synchrotron, and proposed to build one Ø Later Oliphant tried to build one in England but ran out of funds and graduate students Ø In the US, Berkeley (Bevatron) and Brookhaven (Cosmotron) raced to build one n BNL won 1
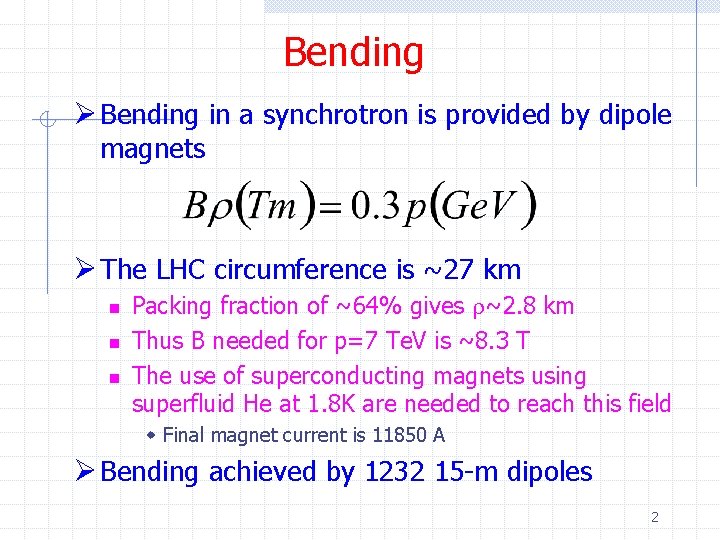
Bending Ø Bending in a synchrotron is provided by dipole magnets Ø The LHC circumference is ~27 km n n n Packing fraction of ~64% gives r~2. 8 km Thus B needed for p=7 Te. V is ~8. 3 T The use of superconducting magnets using superfluid He at 1. 8 K are needed to reach this field w Final magnet current is 11850 A Ø Bending achieved by 1232 15 -m dipoles 2
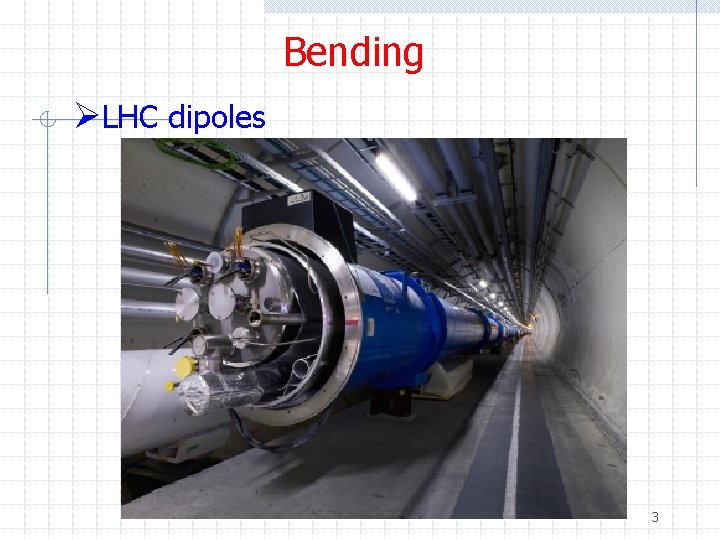
Bending ØLHC dipoles 3
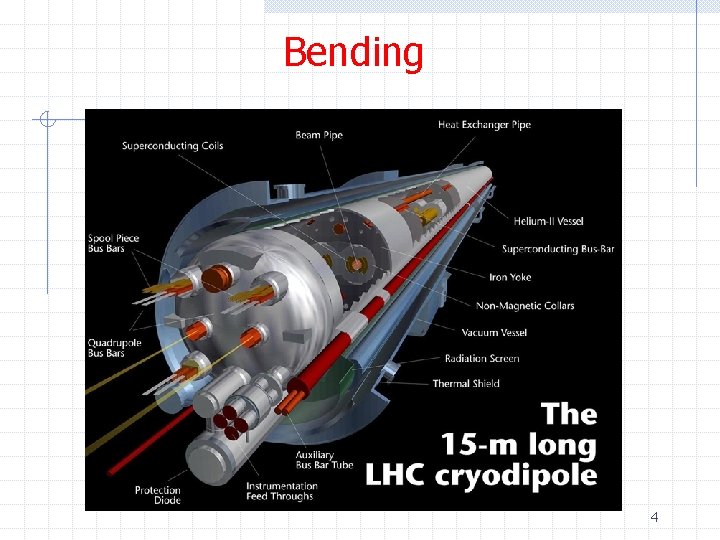
Bending 4
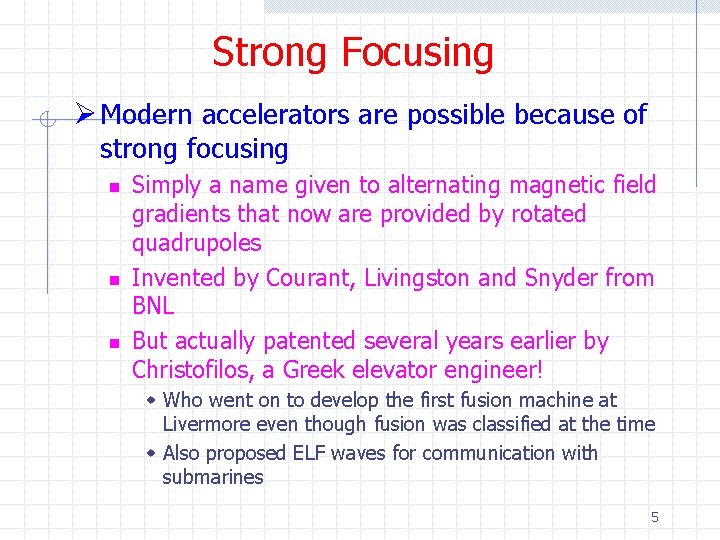
Strong Focusing Ø Modern accelerators are possible because of strong focusing n n n Simply a name given to alternating magnetic field gradients that now are provided by rotated quadrupoles Invented by Courant, Livingston and Snyder from BNL But actually patented several years earlier by Christofilos, a Greek elevator engineer! w Who went on to develop the first fusion machine at Livermore even though fusion was classified at the time w Also proposed ELF waves for communication with submarines 5
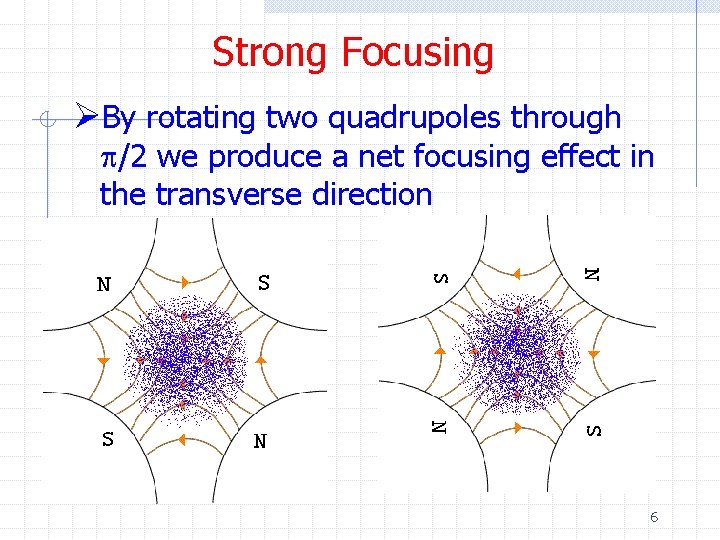
Strong Focusing ØBy rotating two quadrupoles through p/2 we produce a net focusing effect in the transverse direction 6
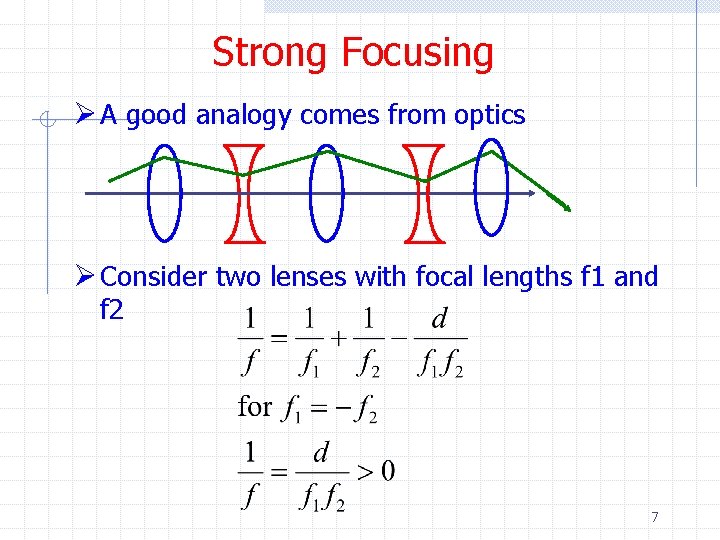
Strong Focusing Ø A good analogy comes from optics Ø Consider two lenses with focal lengths f 1 and f 2 7
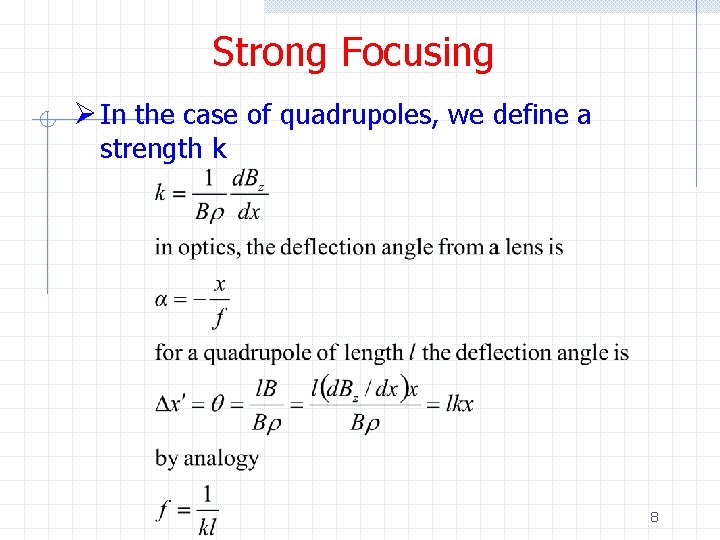
Strong Focusing Ø In the case of quadrupoles, we define a strength k 8
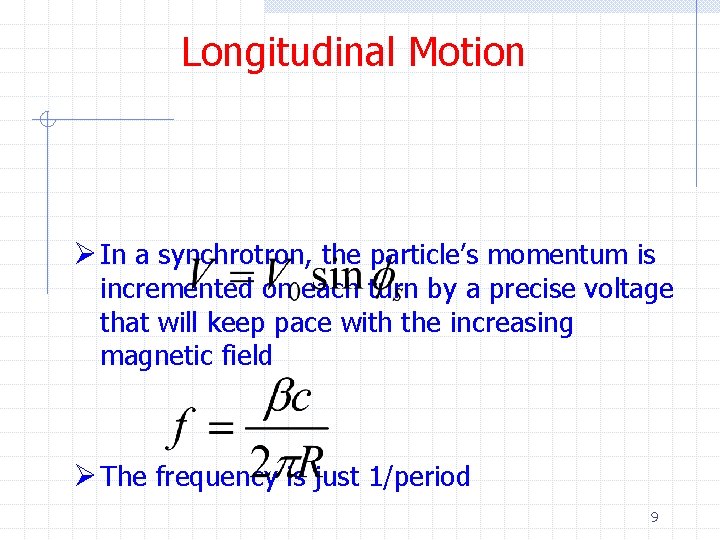
Longitudinal Motion Ø In a synchrotron, the particle’s momentum is incremented on each turn by a precise voltage that will keep pace with the increasing magnetic field Ø The frequency is just 1/period 9
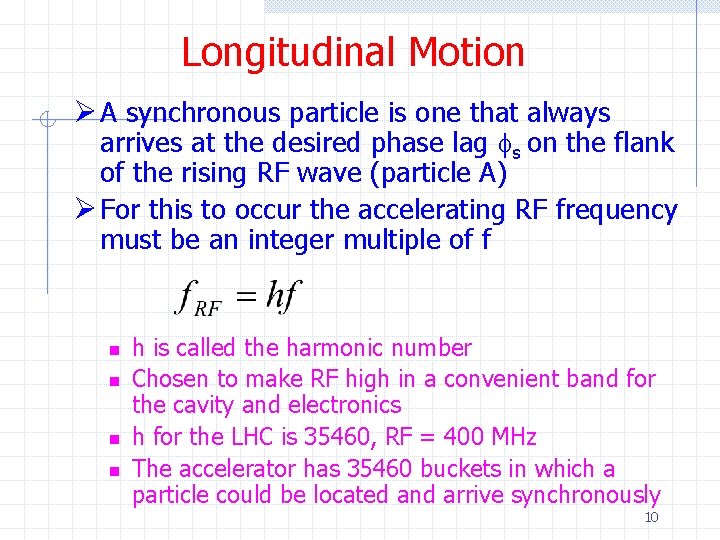
Longitudinal Motion Ø A synchronous particle is one that always arrives at the desired phase lag fs on the flank of the rising RF wave (particle A) Ø For this to occur the accelerating RF frequency must be an integer multiple of f n n h is called the harmonic number Chosen to make RF high in a convenient band for the cavity and electronics h for the LHC is 35460, RF = 400 MHz The accelerator has 35460 buckets in which a particle could be located and arrive synchronously 10
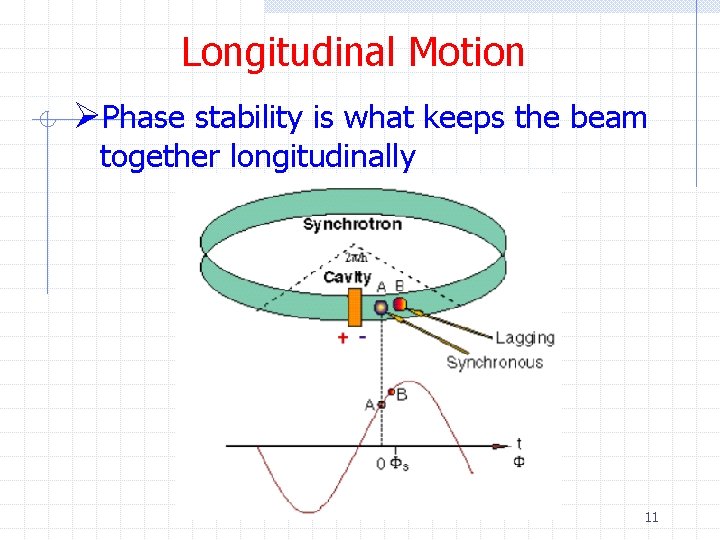
Longitudinal Motion ØPhase stability is what keeps the beam together longitudinally 11
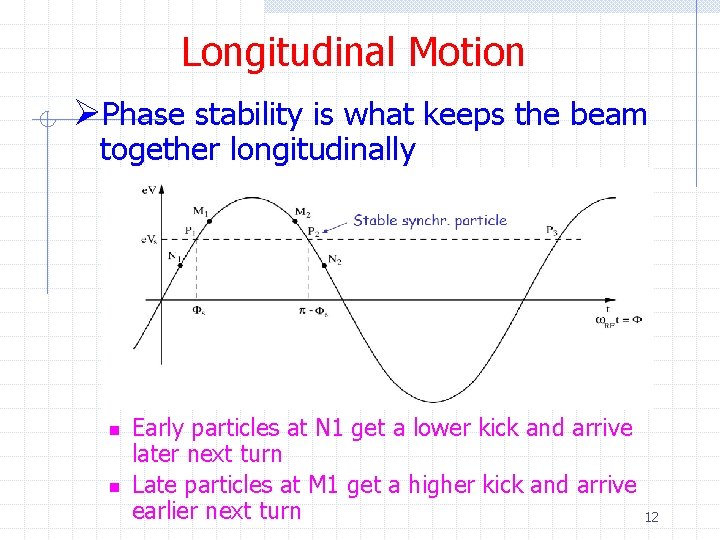
Longitudinal Motion ØPhase stability is what keeps the beam together longitudinally n n Early particles at N 1 get a lower kick and arrive later next turn Late particles at M 1 get a higher kick and arrive earlier next turn 12
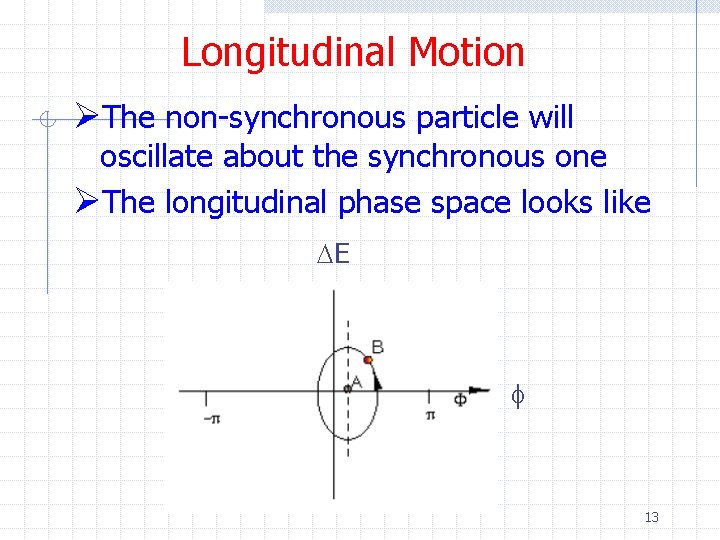
Longitudinal Motion ØThe non-synchronous particle will oscillate about the synchronous one ØThe longitudinal phase space looks like DE f 13
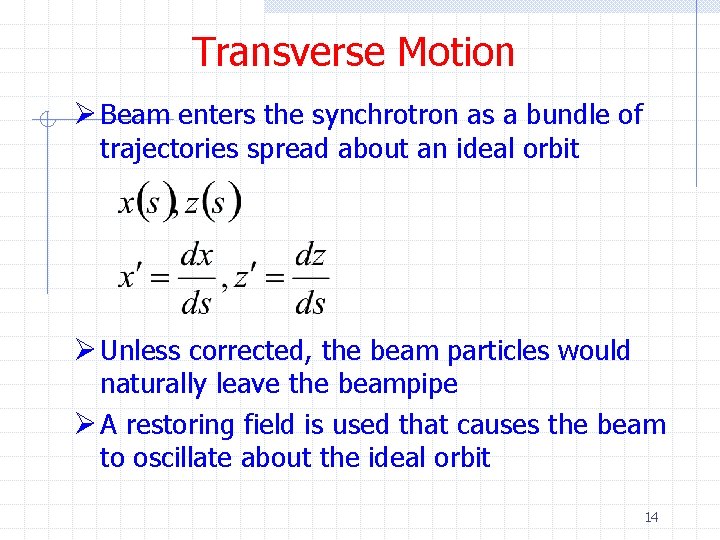
Transverse Motion Ø Beam enters the synchrotron as a bundle of trajectories spread about an ideal orbit Ø Unless corrected, the beam particles would naturally leave the beampipe Ø A restoring field is used that causes the beam to oscillate about the ideal orbit 14
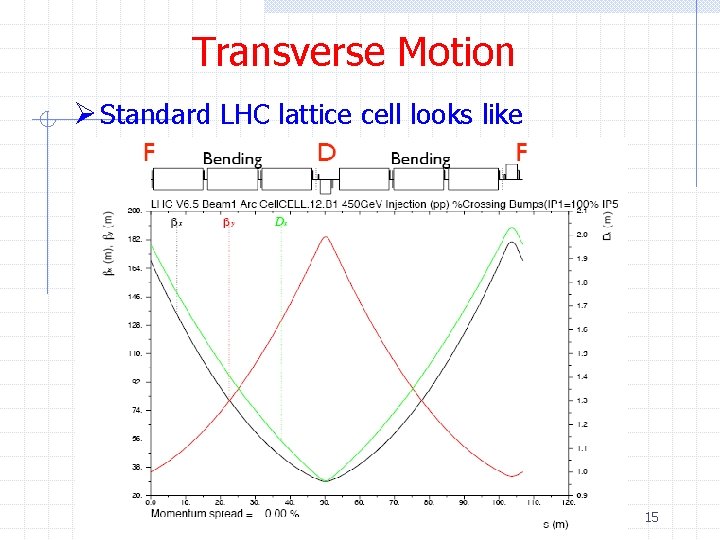
Transverse Motion Ø Standard LHC lattice cell looks like 15
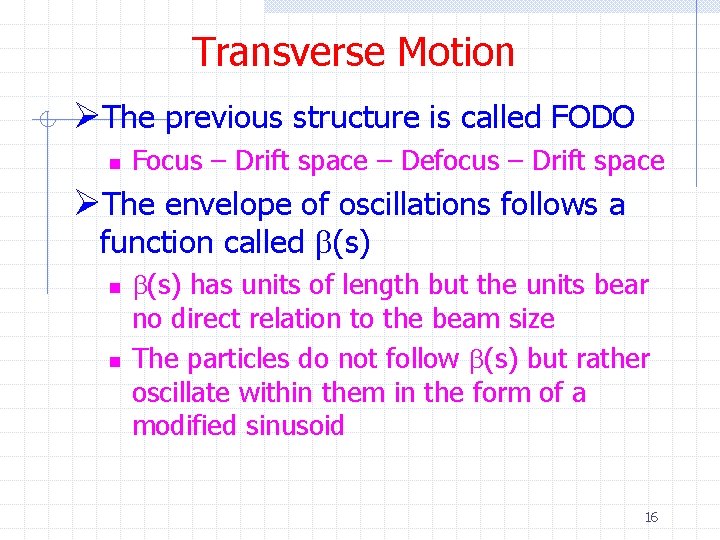
Transverse Motion ØThe previous structure is called FODO n Focus – Drift space – Defocus – Drift space ØThe envelope of oscillations follows a function called b(s) n n b(s) has units of length but the units bear no direct relation to the beam size The particles do not follow b(s) but rather oscillate within them in the form of a modified sinusoid 16
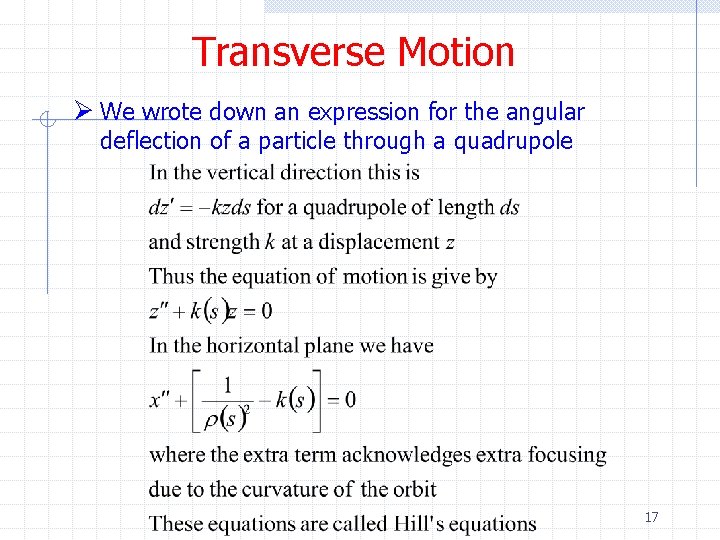
Transverse Motion Ø We wrote down an expression for the angular deflection of a particle through a quadrupole 17
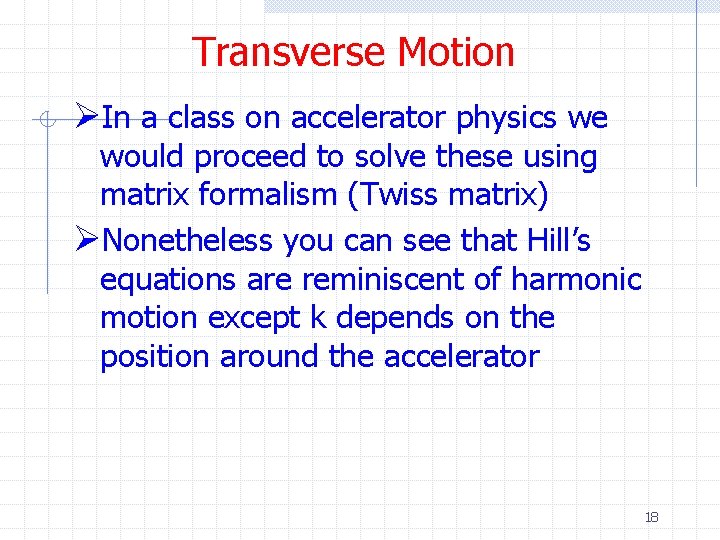
Transverse Motion ØIn a class on accelerator physics we would proceed to solve these using matrix formalism (Twiss matrix) ØNonetheless you can see that Hill’s equations are reminiscent of harmonic motion except k depends on the position around the accelerator 18
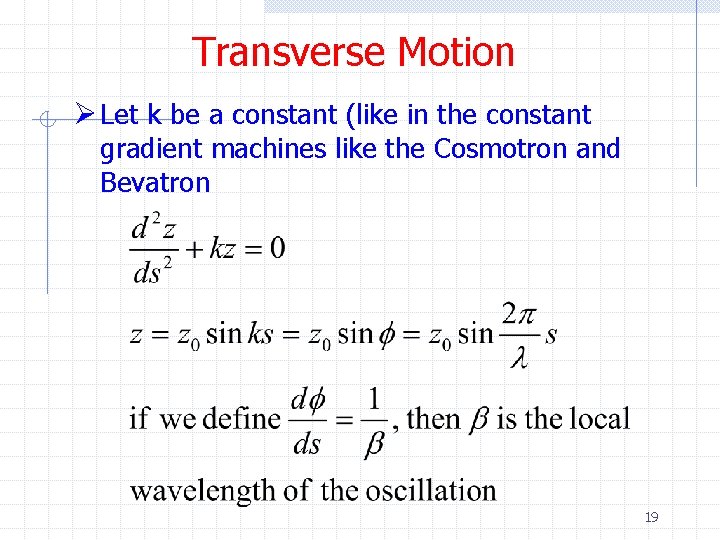
Transverse Motion Ø Let k be a constant (like in the constant gradient machines like the Cosmotron and Bevatron 19
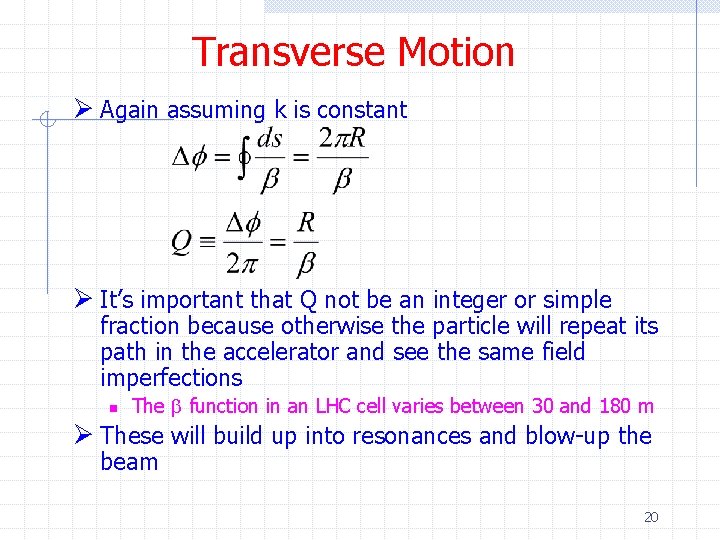
Transverse Motion Ø Again assuming k is constant Ø It’s important that Q not be an integer or simple fraction because otherwise the particle will repeat its path in the accelerator and see the same field imperfections n The b function in an LHC cell varies between 30 and 180 m Ø These will build up into resonances and blow-up the beam 20
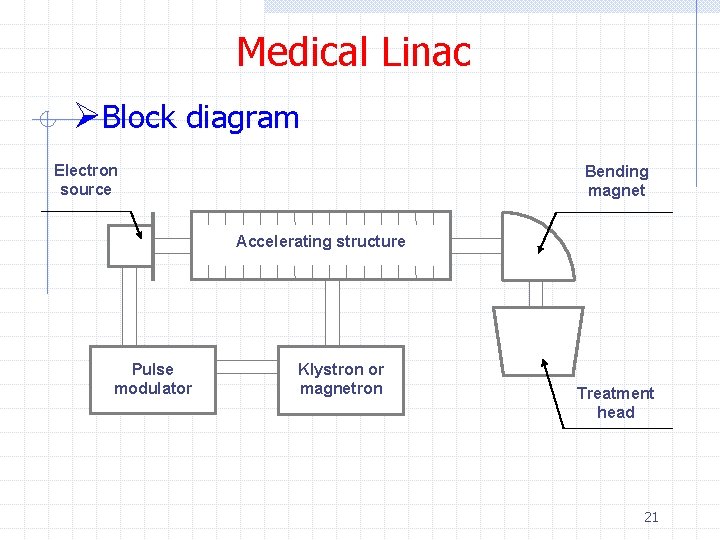
Medical Linac ØBlock diagram Electron source Bending magnet Accelerating structure Pulse modulator Klystron or magnetron Treatment head 21
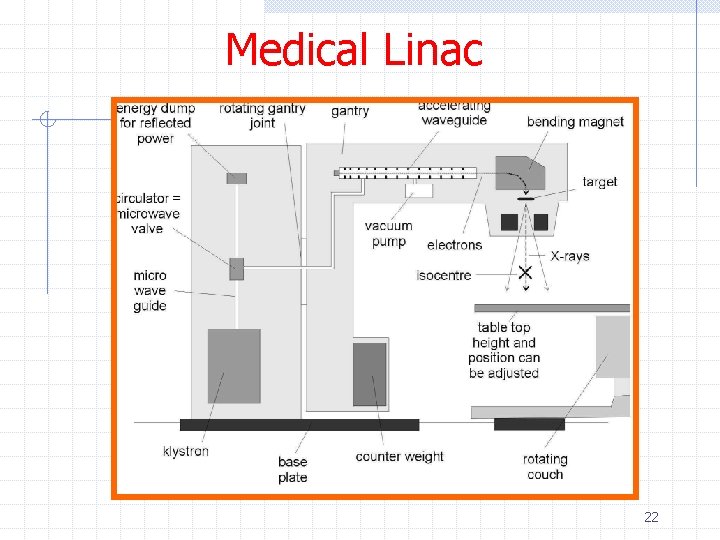
Medical Linac 22
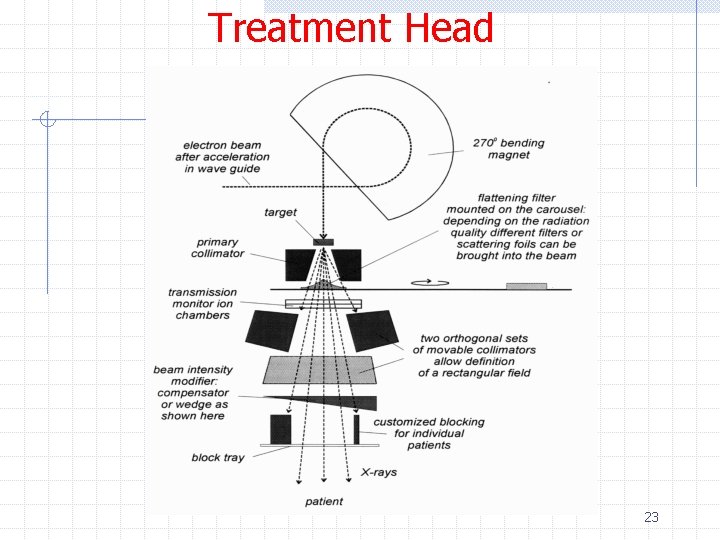
Treatment Head 23
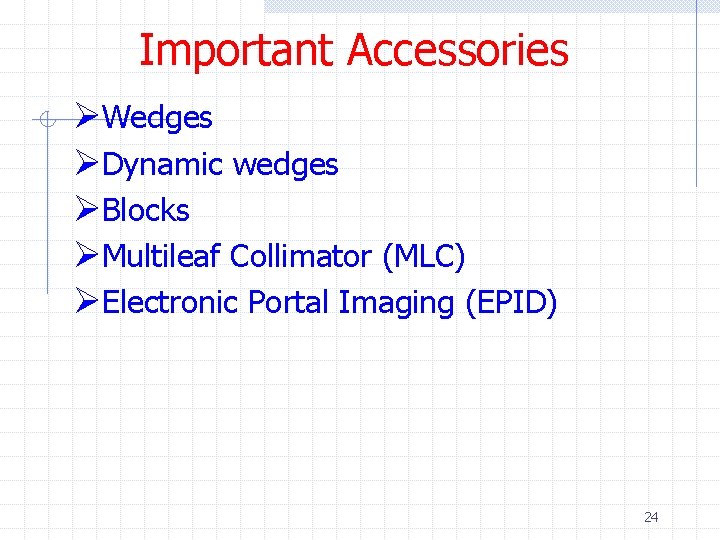
Important Accessories ØWedges ØDynamic wedges ØBlocks ØMultileaf Collimator (MLC) ØElectronic Portal Imaging (EPID) 24
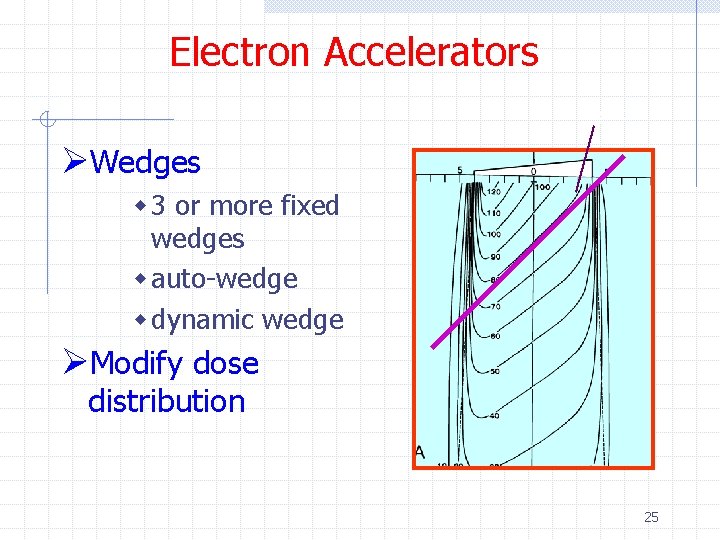
Electron Accelerators angle ØWedges w 3 or more fixed wedges w auto-wedge w dynamic wedge ØModify dose distribution 25
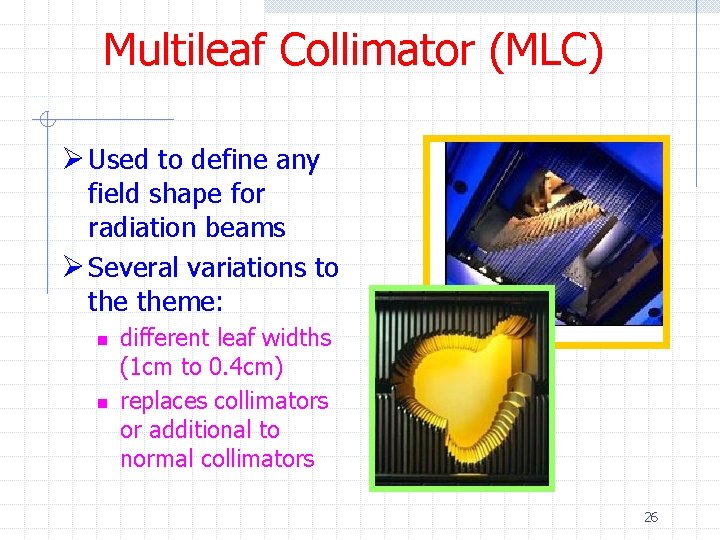
Multileaf Collimator (MLC) Ø Used to define any field shape for radiation beams Ø Several variations to theme: n n different leaf widths (1 cm to 0. 4 cm) replaces collimators or additional to normal collimators 26
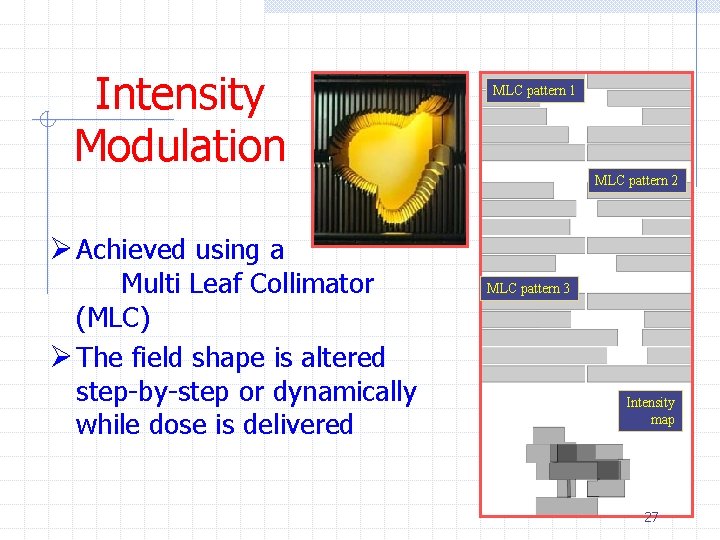
Intensity Modulation MLC pattern 1 MLC pattern 2 Ø Achieved using a Multi Leaf Collimator (MLC) Ø The field shape is altered step-by-step or dynamically while dose is delivered MLC pattern 3 Intensity map 27
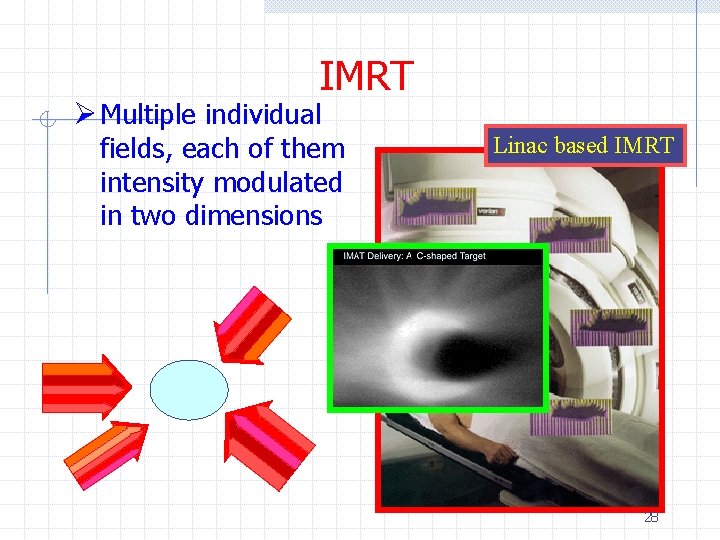
IMRT Ø Multiple individual fields, each of them intensity modulated in two dimensions Linac based IMRT 28
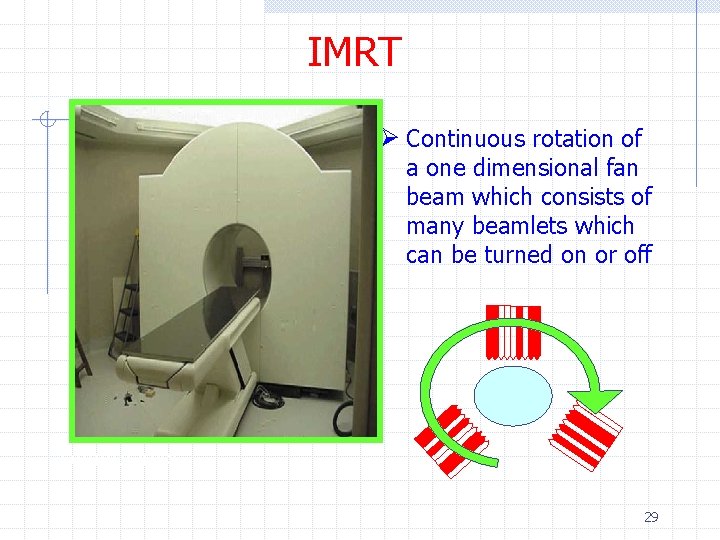
IMRT Ø Continuous rotation of a one dimensional fan beam which consists of many beamlets which can be turned on or off Tomotherapy 29
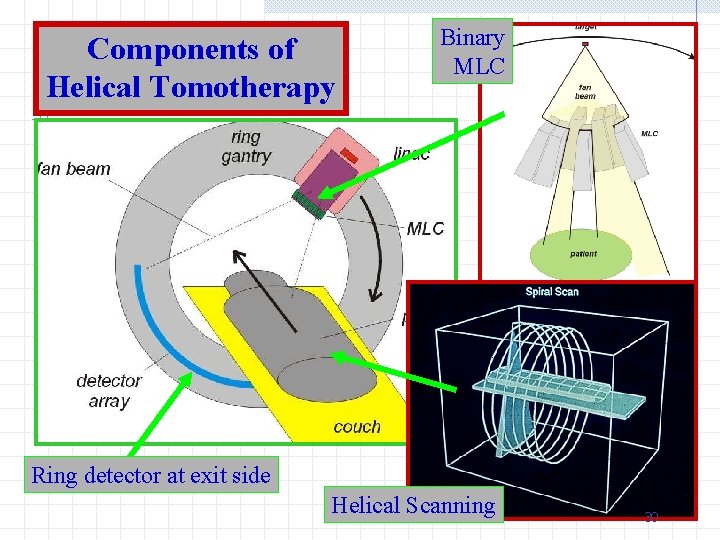
Components of Helical Tomotherapy Binary MLC Ring detector at exit side Helical Scanning 30
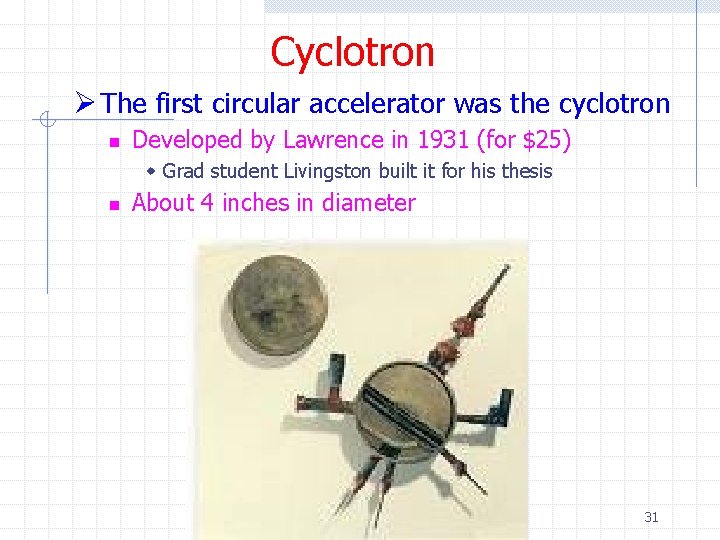
Cyclotron Ø The first circular accelerator was the cyclotron n Developed by Lawrence in 1931 (for $25) w Grad student Livingston built it for his thesis n About 4 inches in diameter 31
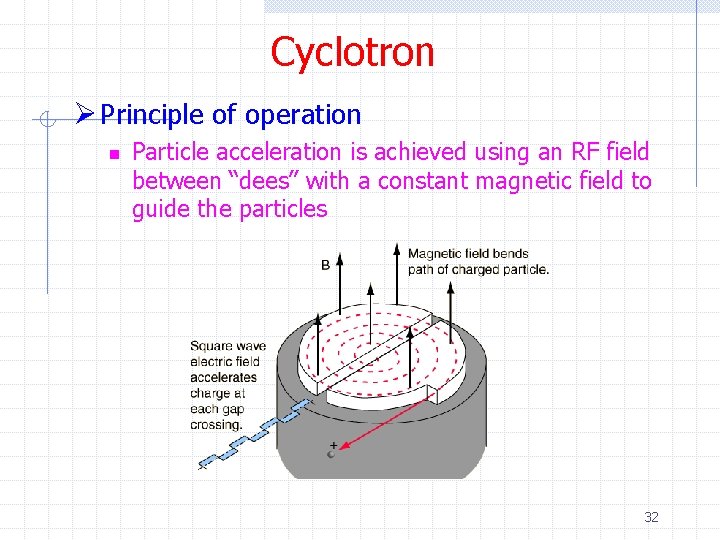
Cyclotron Ø Principle of operation n Particle acceleration is achieved using an RF field between “dees” with a constant magnetic field to guide the particles 32
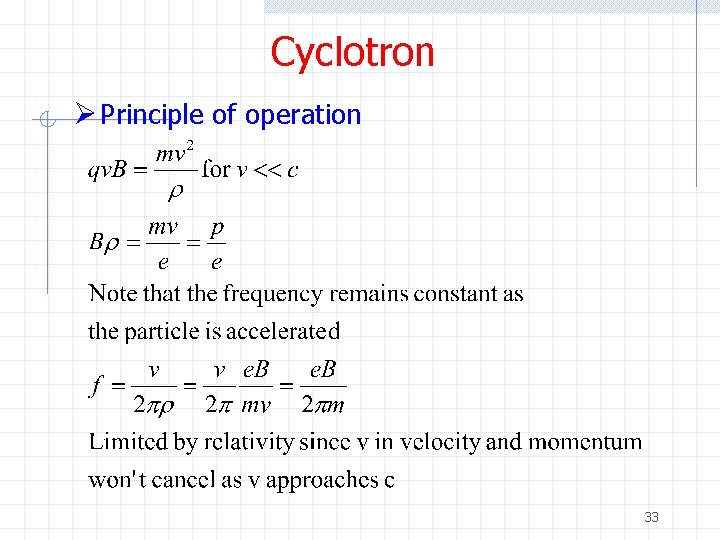
Cyclotron Ø Principle of operation 33
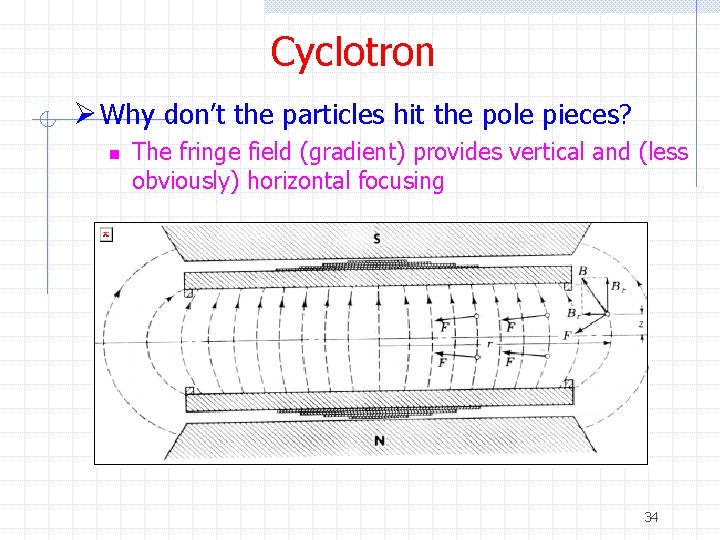
Cyclotron Ø Why don’t the particles hit the pole pieces? n The fringe field (gradient) provides vertical and (less obviously) horizontal focusing 34
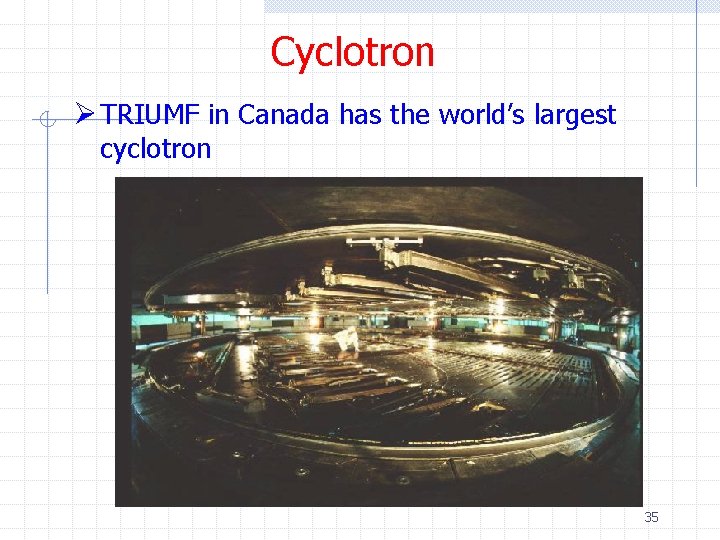
Cyclotron Ø TRIUMF in Canada has the world’s largest cyclotron 35
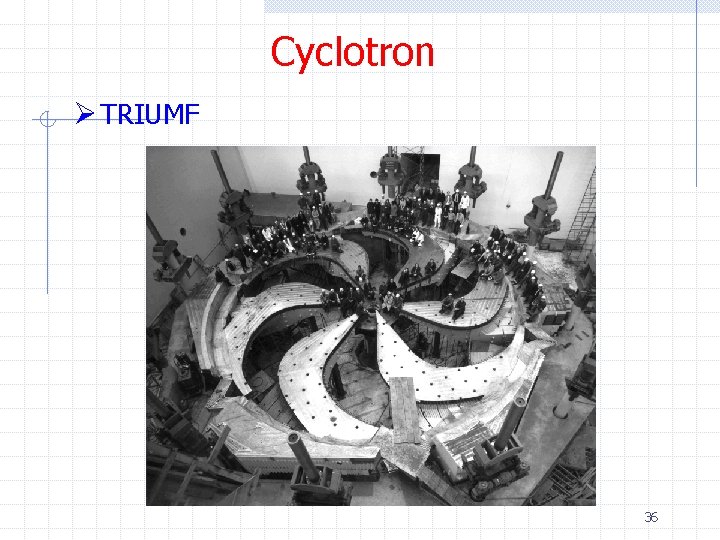
Cyclotron Ø TRIUMF 36
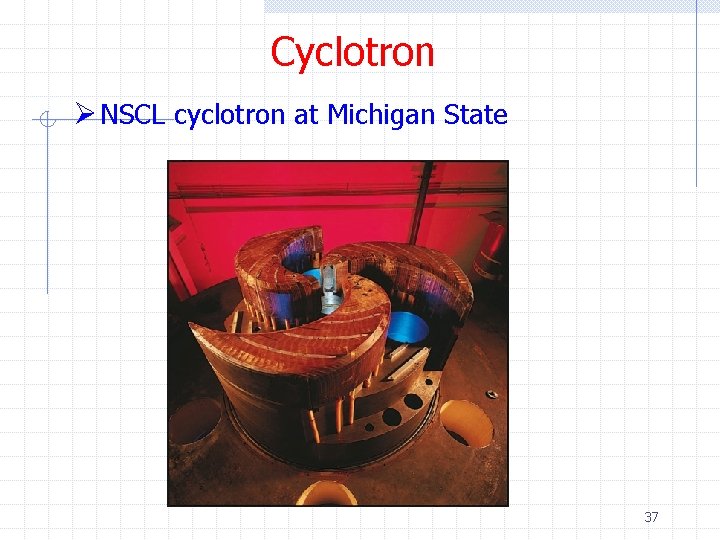
Cyclotron Ø NSCL cyclotron at Michigan State 37
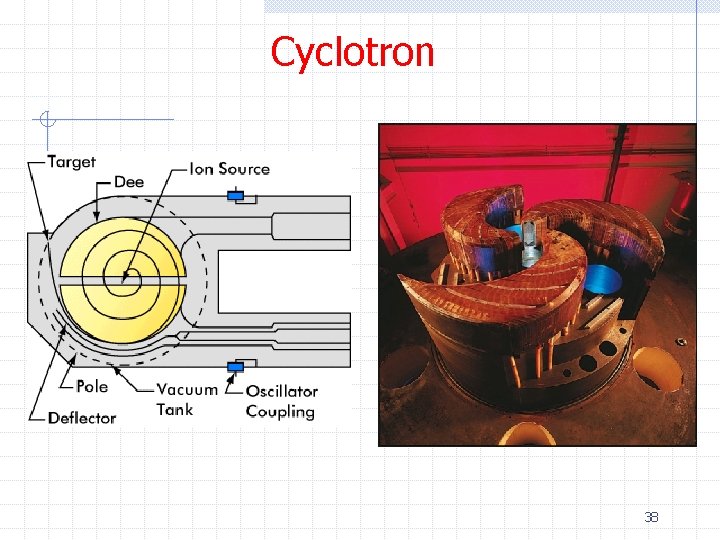
Cyclotron 38
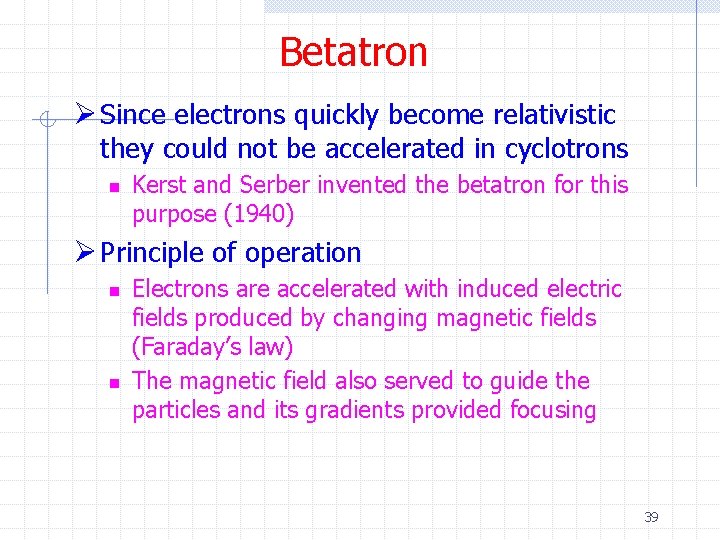
Betatron Ø Since electrons quickly become relativistic they could not be accelerated in cyclotrons n Kerst and Serber invented the betatron for this purpose (1940) Ø Principle of operation n n Electrons are accelerated with induced electric fields produced by changing magnetic fields (Faraday’s law) The magnetic field also served to guide the particles and its gradients provided focusing 39
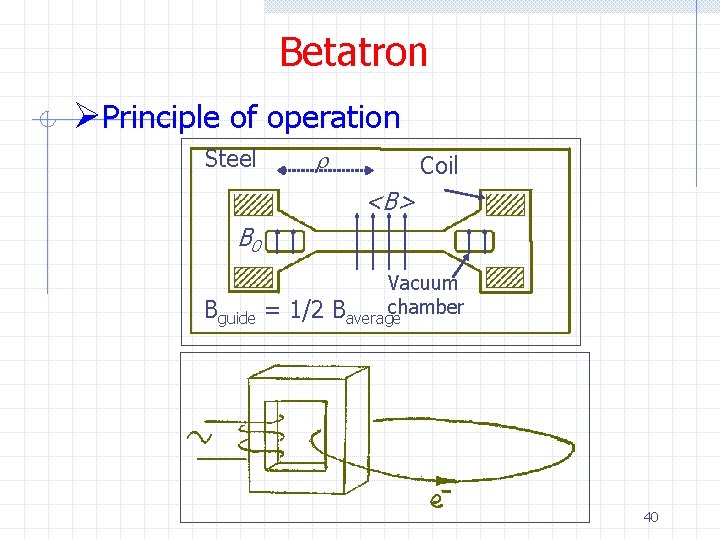
Betatron ØPrinciple of operation Steel r Coil <B> B 0 Vacuum chamber Bguide = 1/2 Baverage 40
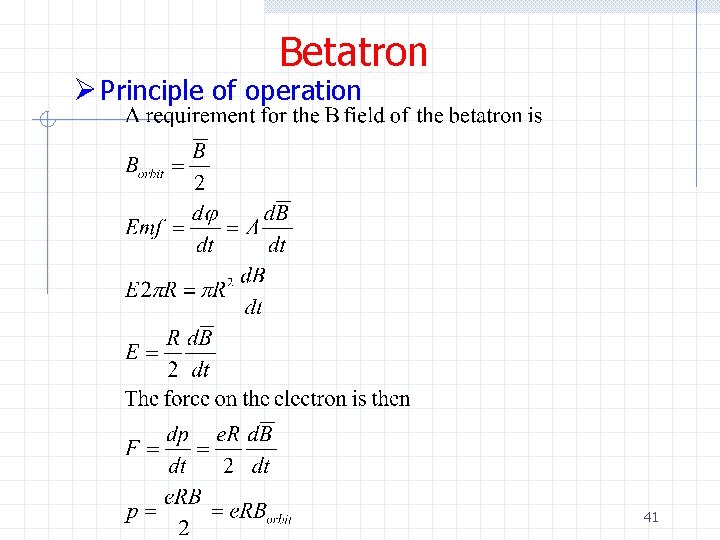
Betatron Ø Principle of operation 41
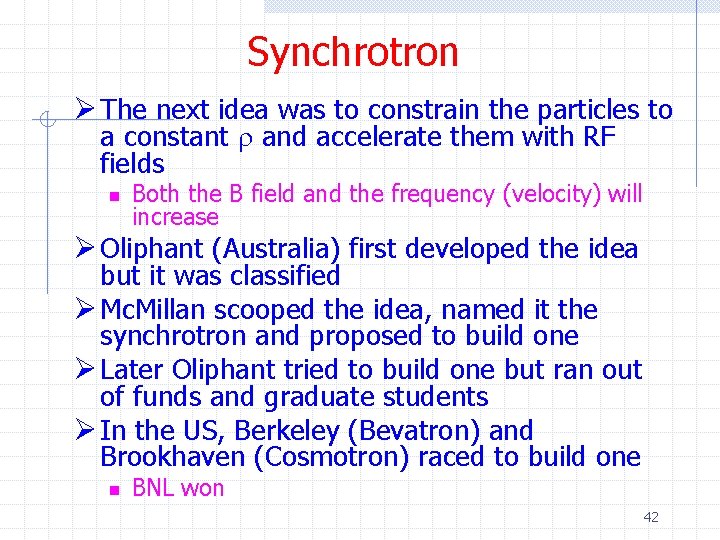
Synchrotron Ø The next idea was to constrain the particles to a constant r and accelerate them with RF fields n Both the B field and the frequency (velocity) will increase Ø Oliphant (Australia) first developed the idea but it was classified Ø Mc. Millan scooped the idea, named it the synchrotron and proposed to build one Ø Later Oliphant tried to build one but ran out of funds and graduate students Ø In the US, Berkeley (Bevatron) and Brookhaven (Cosmotron) raced to build one n BNL won 42
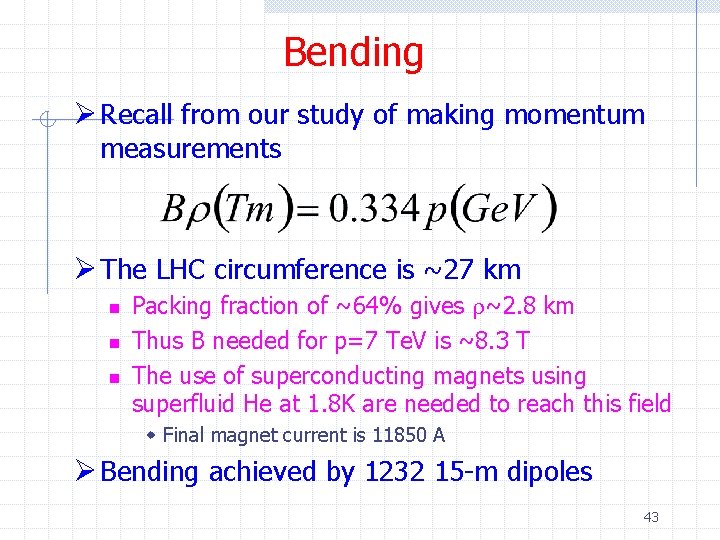
Bending Ø Recall from our study of making momentum measurements Ø The LHC circumference is ~27 km n n n Packing fraction of ~64% gives r~2. 8 km Thus B needed for p=7 Te. V is ~8. 3 T The use of superconducting magnets using superfluid He at 1. 8 K are needed to reach this field w Final magnet current is 11850 A Ø Bending achieved by 1232 15 -m dipoles 43
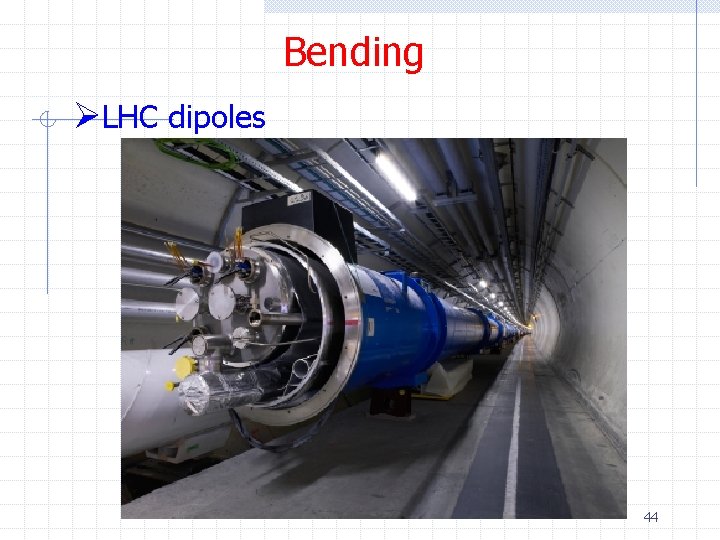
Bending ØLHC dipoles 44
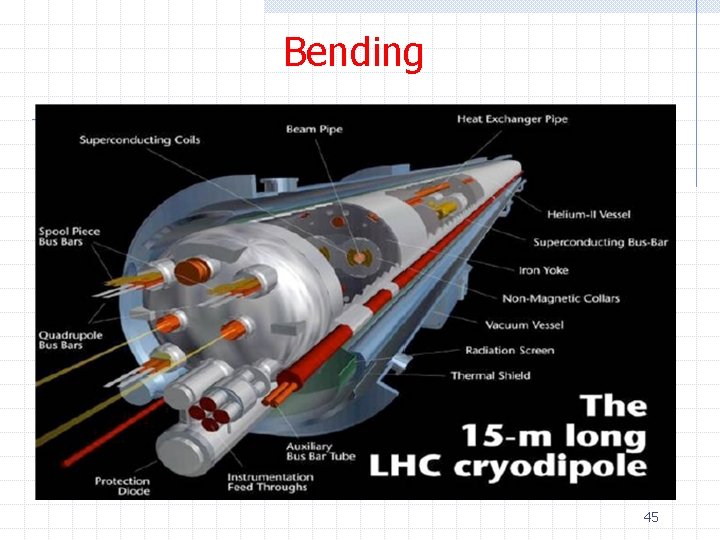
Bending 45
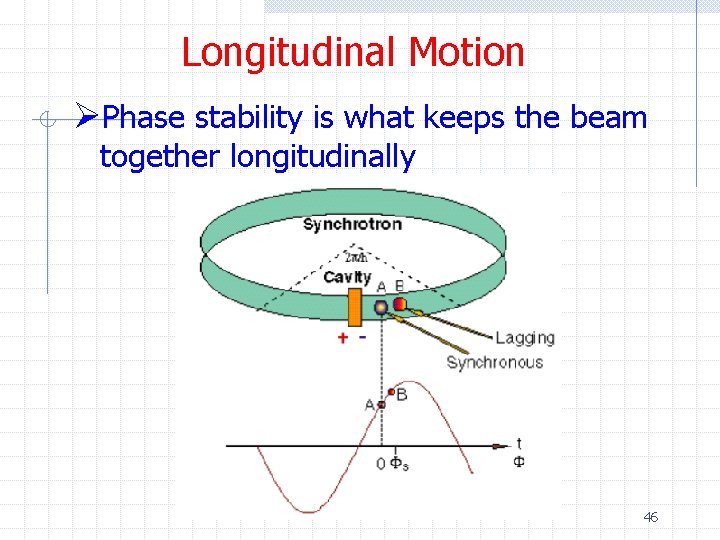
Longitudinal Motion ØPhase stability is what keeps the beam together longitudinally 46
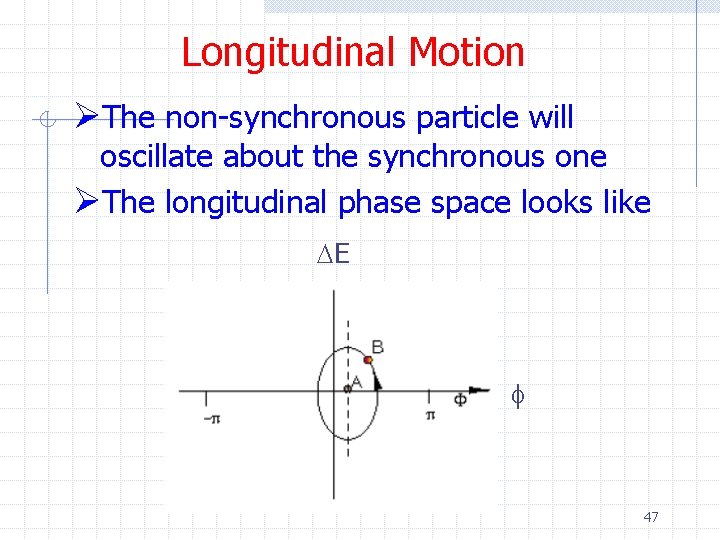
Longitudinal Motion ØThe non-synchronous particle will oscillate about the synchronous one ØThe longitudinal phase space looks like DE f 47
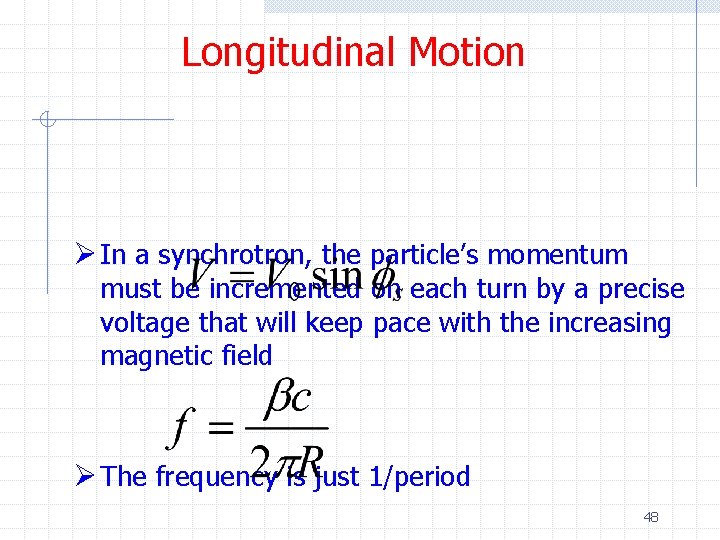
Longitudinal Motion Ø In a synchrotron, the particle’s momentum must be incremented on each turn by a precise voltage that will keep pace with the increasing magnetic field Ø The frequency is just 1/period 48
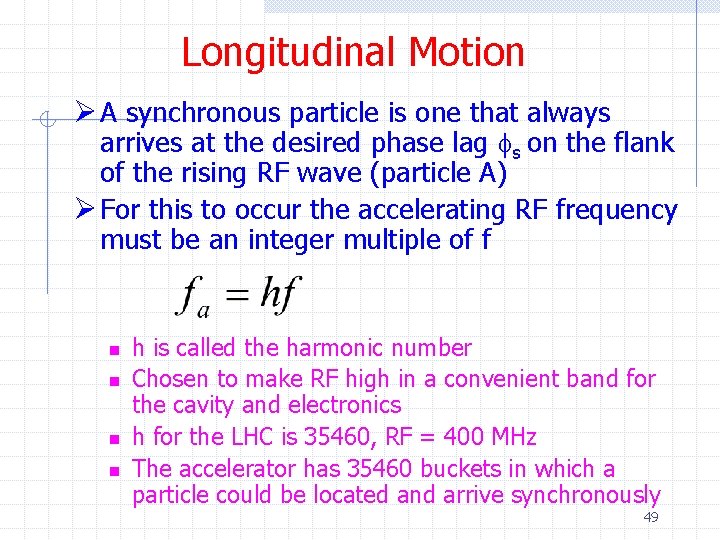
Longitudinal Motion Ø A synchronous particle is one that always arrives at the desired phase lag fs on the flank of the rising RF wave (particle A) Ø For this to occur the accelerating RF frequency must be an integer multiple of f n n h is called the harmonic number Chosen to make RF high in a convenient band for the cavity and electronics h for the LHC is 35460, RF = 400 MHz The accelerator has 35460 buckets in which a particle could be located and arrive synchronously 49
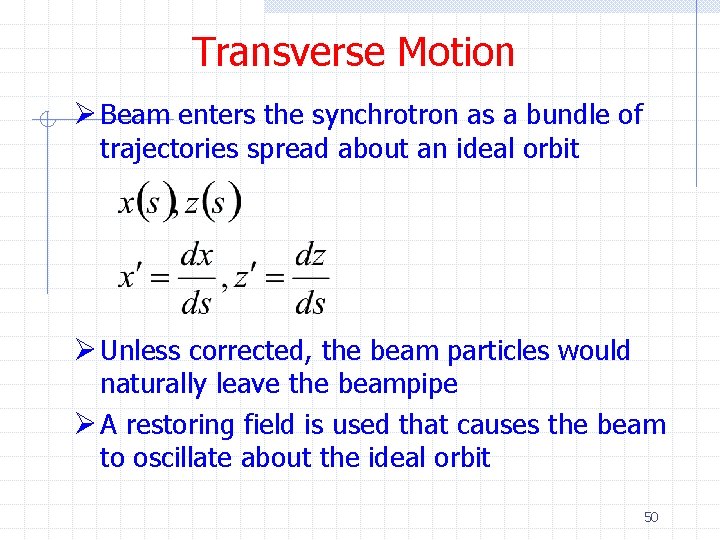
Transverse Motion Ø Beam enters the synchrotron as a bundle of trajectories spread about an ideal orbit Ø Unless corrected, the beam particles would naturally leave the beampipe Ø A restoring field is used that causes the beam to oscillate about the ideal orbit 50
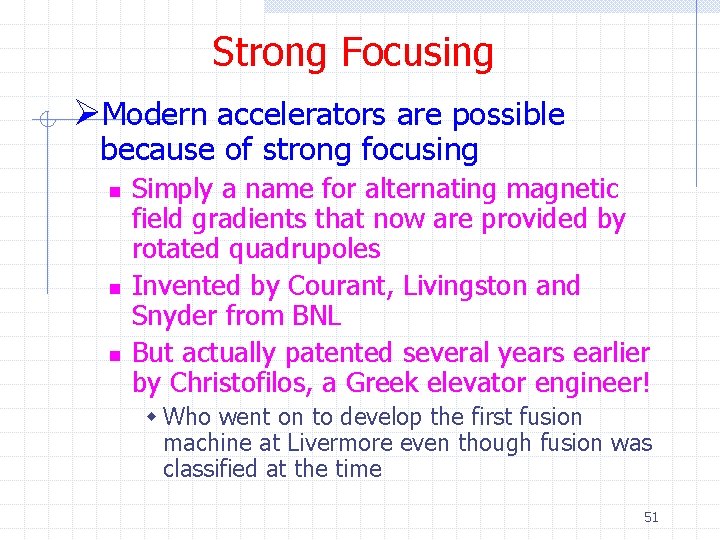
Strong Focusing ØModern accelerators are possible because of strong focusing n n n Simply a name for alternating magnetic field gradients that now are provided by rotated quadrupoles Invented by Courant, Livingston and Snyder from BNL But actually patented several years earlier by Christofilos, a Greek elevator engineer! w Who went on to develop the first fusion machine at Livermore even though fusion was classified at the time 51
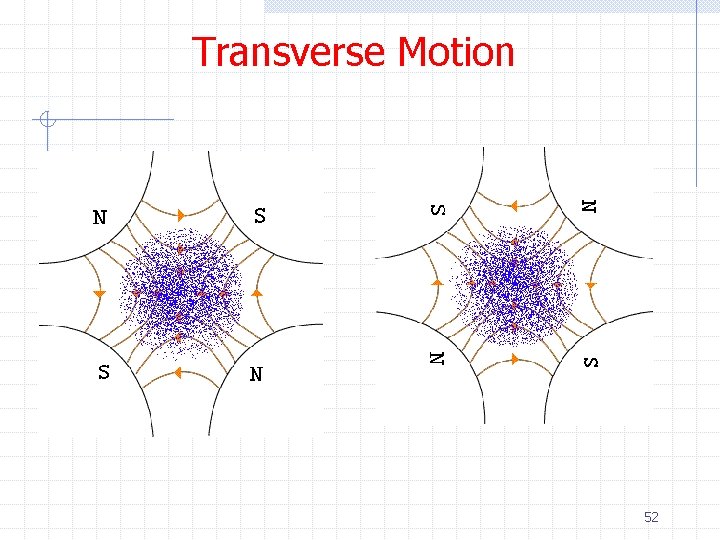
Transverse Motion 52
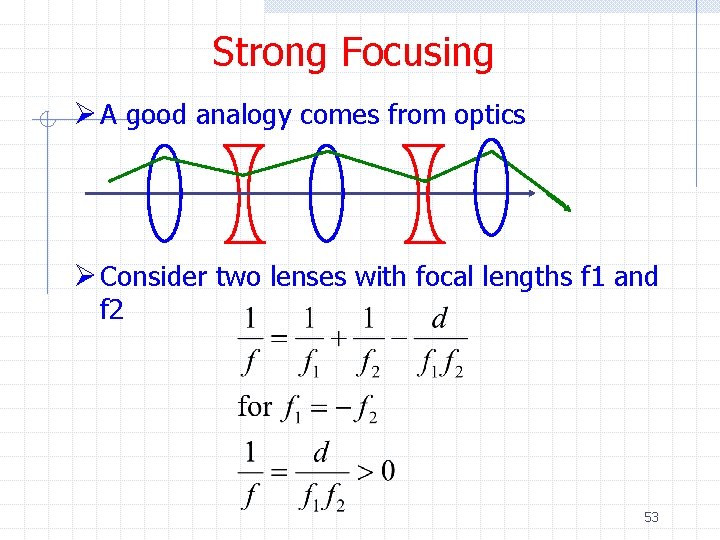
Strong Focusing Ø A good analogy comes from optics Ø Consider two lenses with focal lengths f 1 and f 2 53
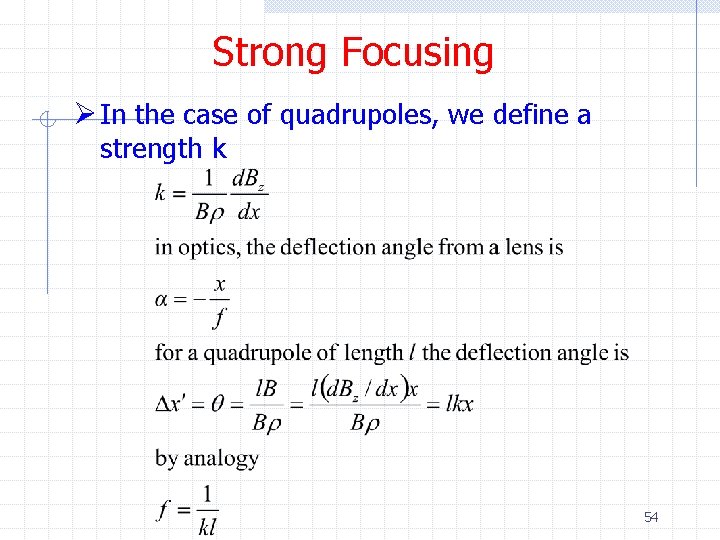
Strong Focusing Ø In the case of quadrupoles, we define a strength k 54
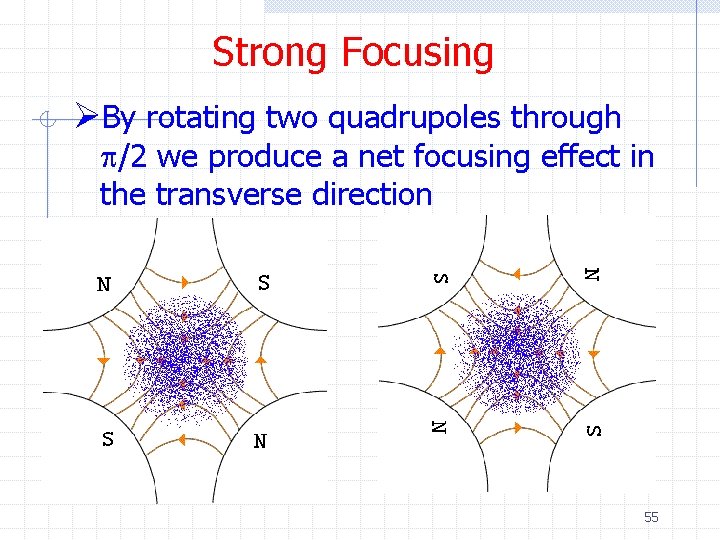
Strong Focusing ØBy rotating two quadrupoles through p/2 we produce a net focusing effect in the transverse direction 55
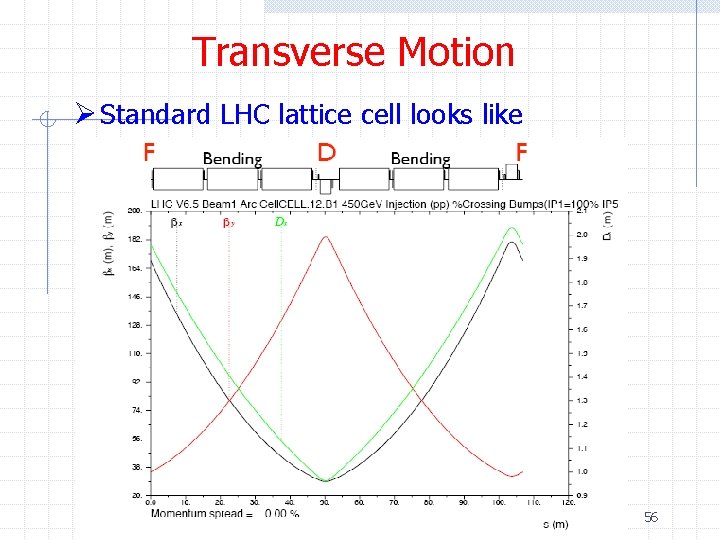
Transverse Motion Ø Standard LHC lattice cell looks like 56
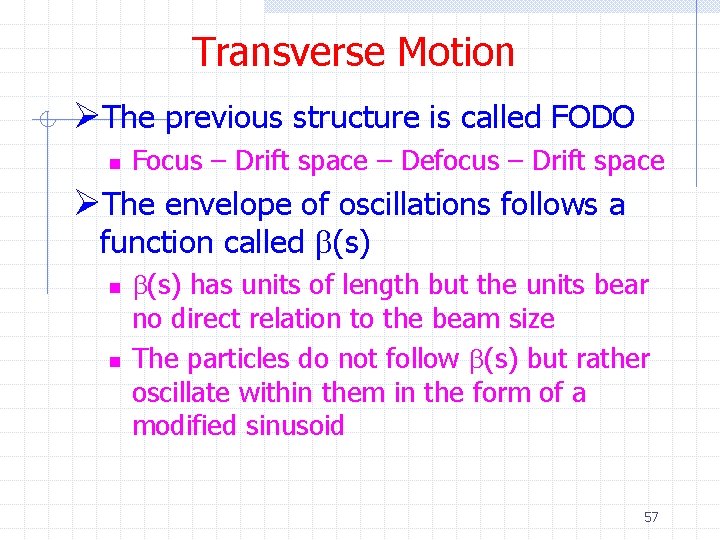
Transverse Motion ØThe previous structure is called FODO n Focus – Drift space – Defocus – Drift space ØThe envelope of oscillations follows a function called b(s) n n b(s) has units of length but the units bear no direct relation to the beam size The particles do not follow b(s) but rather oscillate within them in the form of a modified sinusoid 57
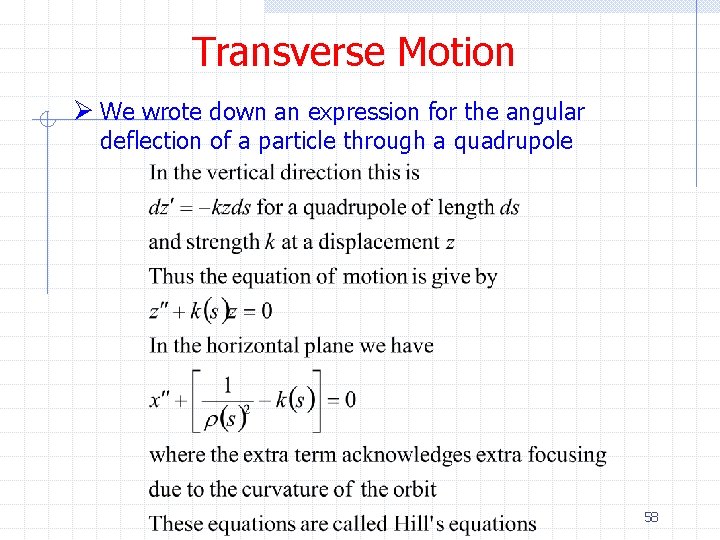
Transverse Motion Ø We wrote down an expression for the angular deflection of a particle through a quadrupole 58
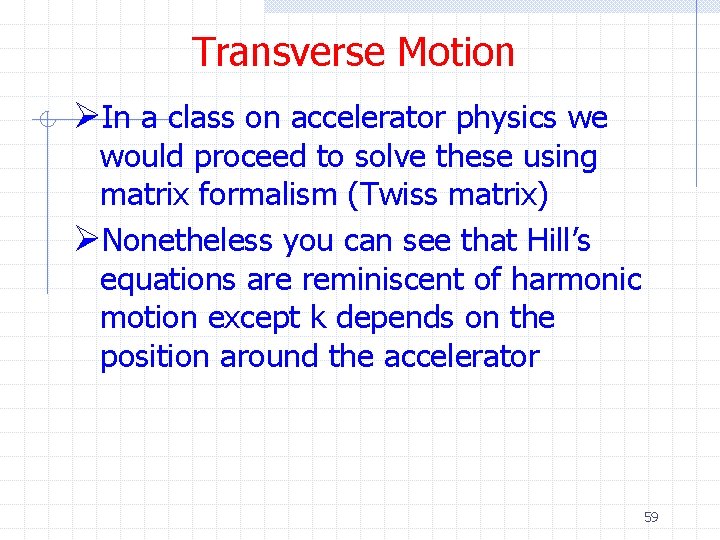
Transverse Motion ØIn a class on accelerator physics we would proceed to solve these using matrix formalism (Twiss matrix) ØNonetheless you can see that Hill’s equations are reminiscent of harmonic motion except k depends on the position around the accelerator 59
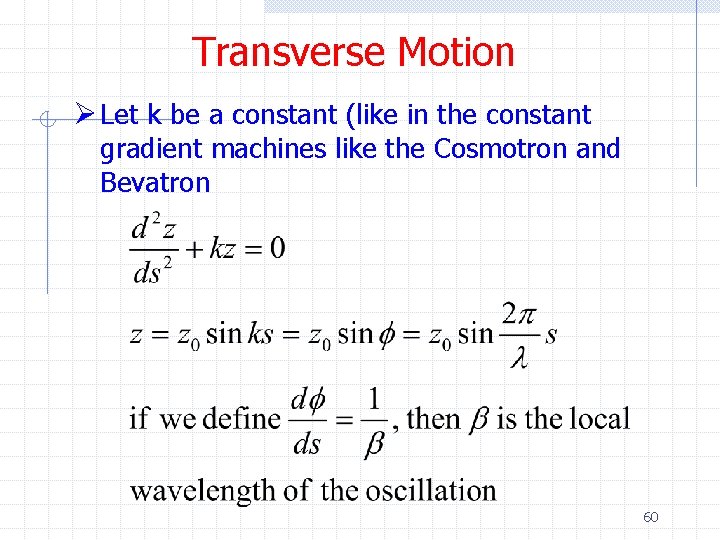
Transverse Motion Ø Let k be a constant (like in the constant gradient machines like the Cosmotron and Bevatron 60
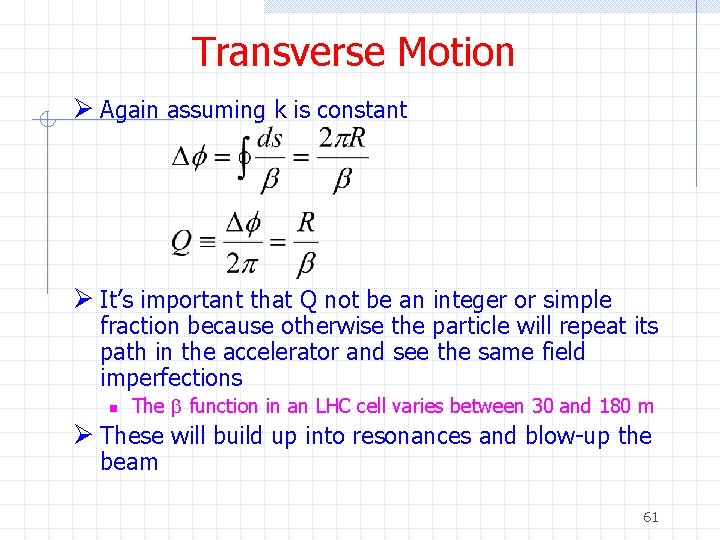
Transverse Motion Ø Again assuming k is constant Ø It’s important that Q not be an integer or simple fraction because otherwise the particle will repeat its path in the accelerator and see the same field imperfections n The b function in an LHC cell varies between 30 and 180 m Ø These will build up into resonances and blow-up the beam 61
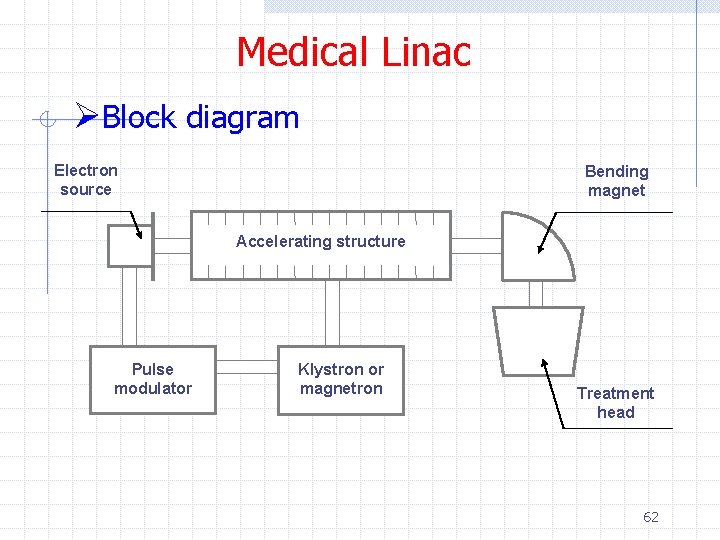
Medical Linac ØBlock diagram Electron source Bending magnet Accelerating structure Pulse modulator Klystron or magnetron Treatment head 62
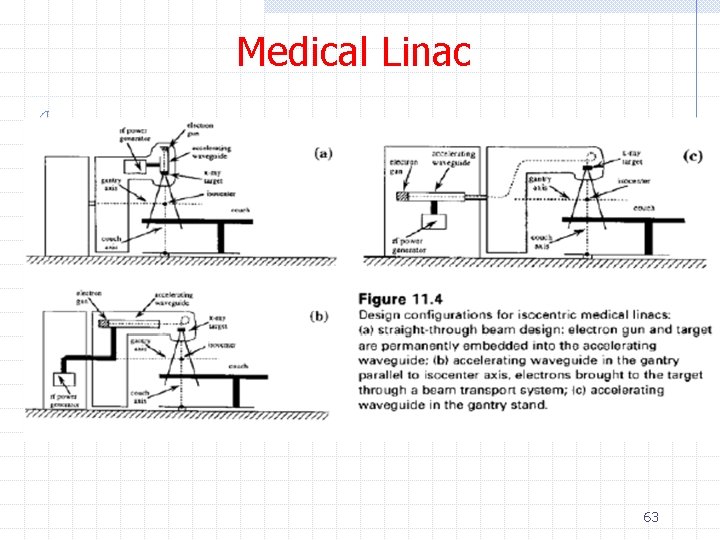
Medical Linac 63
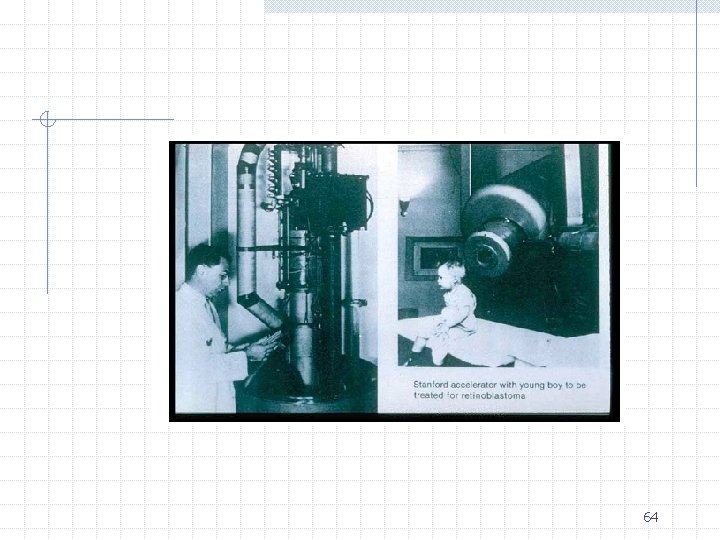
64
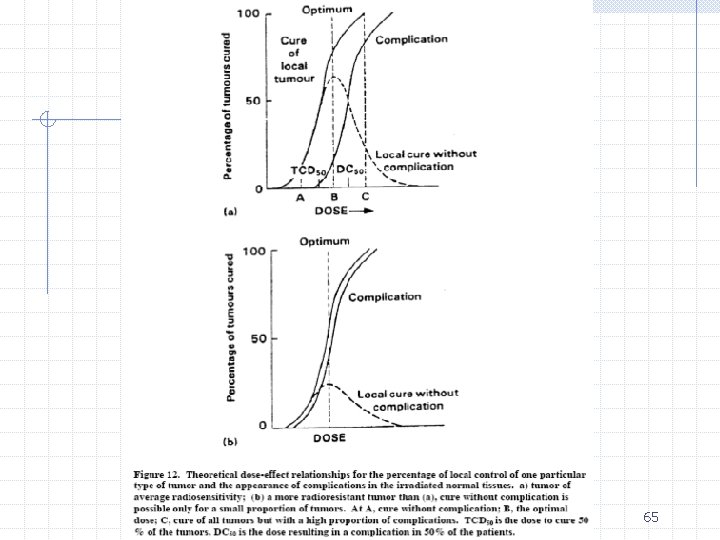
65
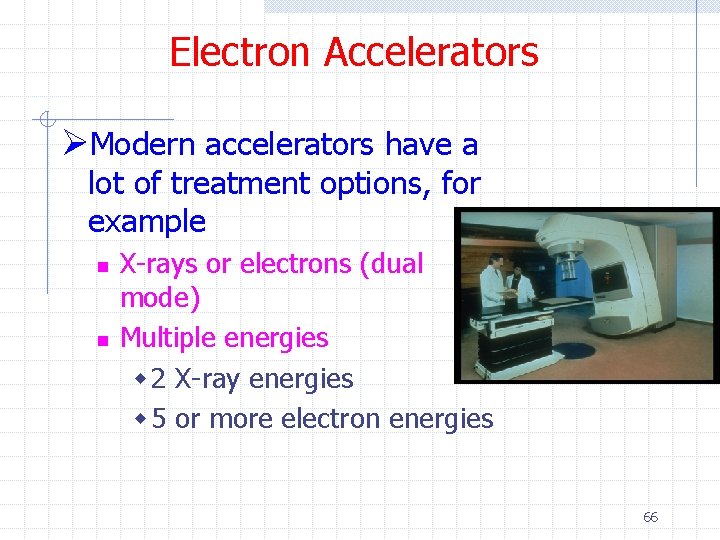
Electron Accelerators ØModern accelerators have a lot of treatment options, for example n n X-rays or electrons (dual mode) Multiple energies w 2 X-ray energies w 5 or more electron energies 66
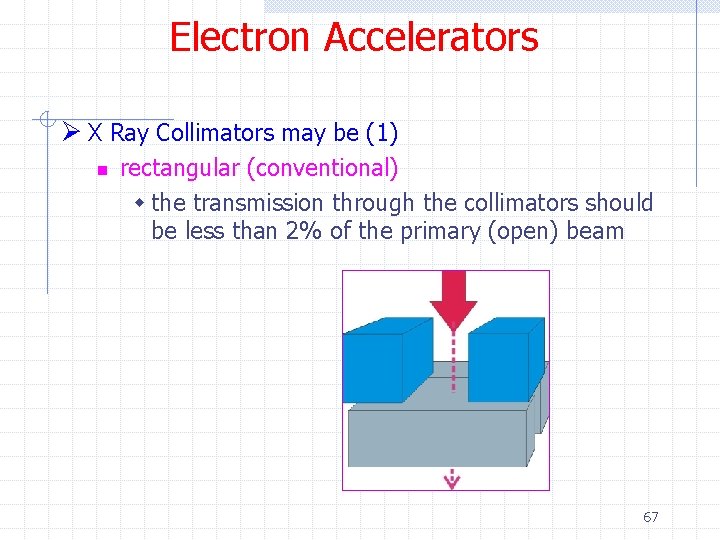
Electron Accelerators Ø X Ray Collimators may be (1) n rectangular (conventional) w the transmission through the collimators should be less than 2% of the primary (open) beam 67
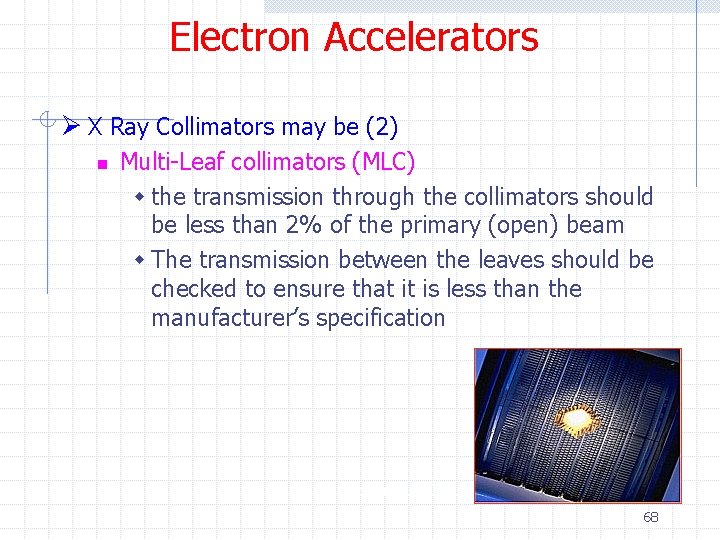
Electron Accelerators Ø X Ray Collimators may be (2) n Multi-Leaf collimators (MLC) w the transmission through the collimators should be less than 2% of the primary (open) beam w The transmission between the leaves should be checked to ensure that it is less than the manufacturer’s specification Siemens MLC 68
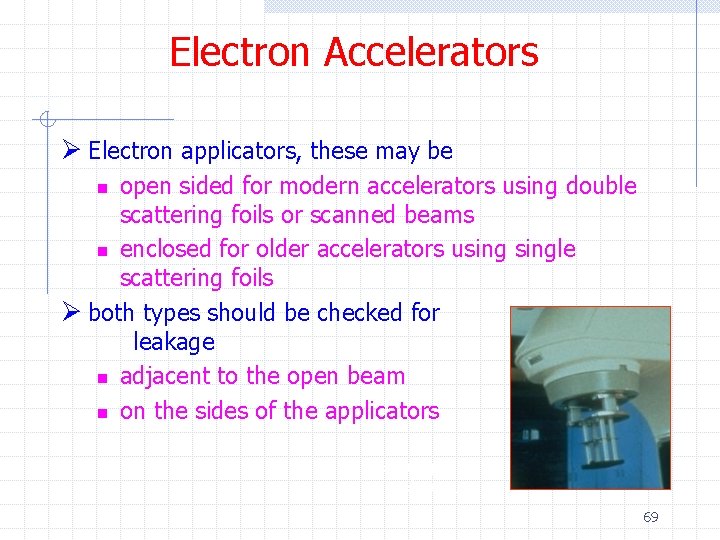
Electron Accelerators Ø Electron applicators, these may be open sided for modern accelerators using double scattering foils or scanned beams n enclosed for older accelerators usingle scattering foils Ø both types should be checked for leakage n adjacent to the open beam n on the sides of the applicators n Varian open sided electron cone 69
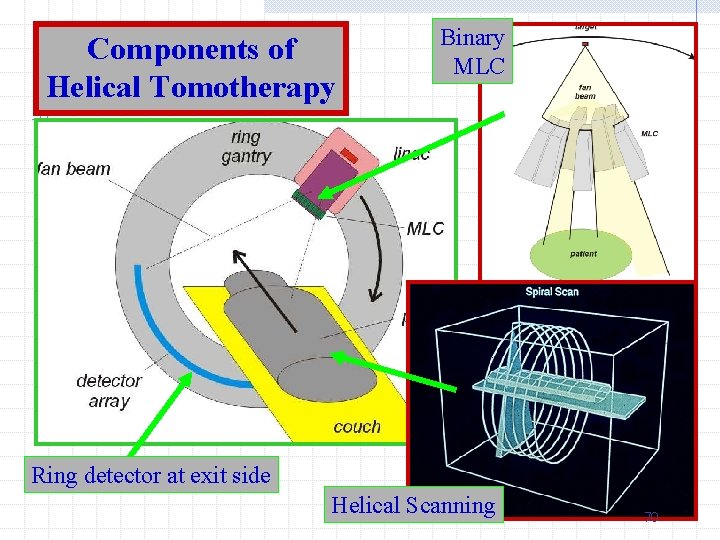
Components of Helical Tomotherapy Binary MLC Ring detector at exit side Helical Scanning 70
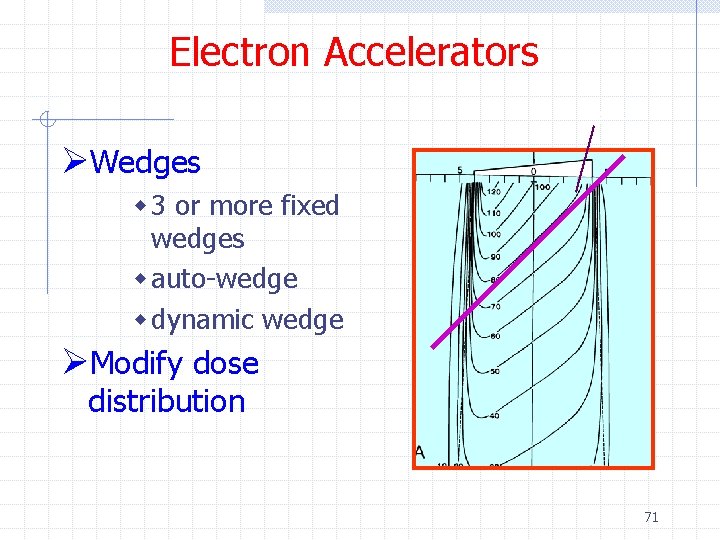
Electron Accelerators angle ØWedges w 3 or more fixed wedges w auto-wedge w dynamic wedge ØModify dose distribution 71
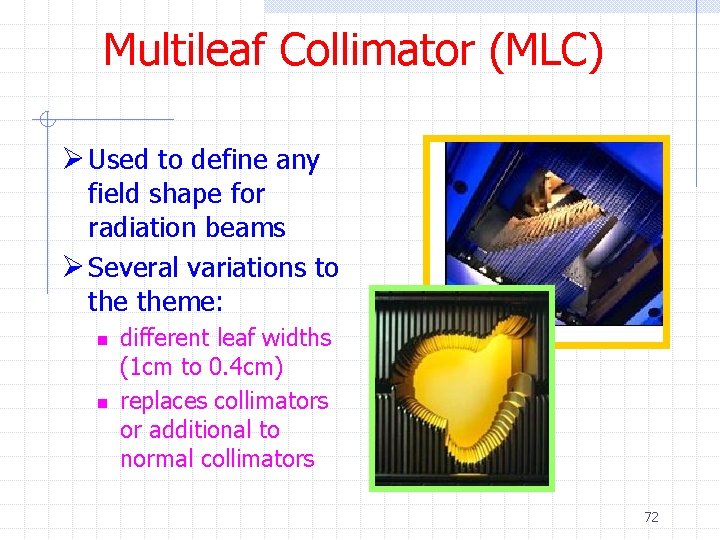
Multileaf Collimator (MLC) Ø Used to define any field shape for radiation beams Ø Several variations to theme: n n different leaf widths (1 cm to 0. 4 cm) replaces collimators or additional to normal collimators 72
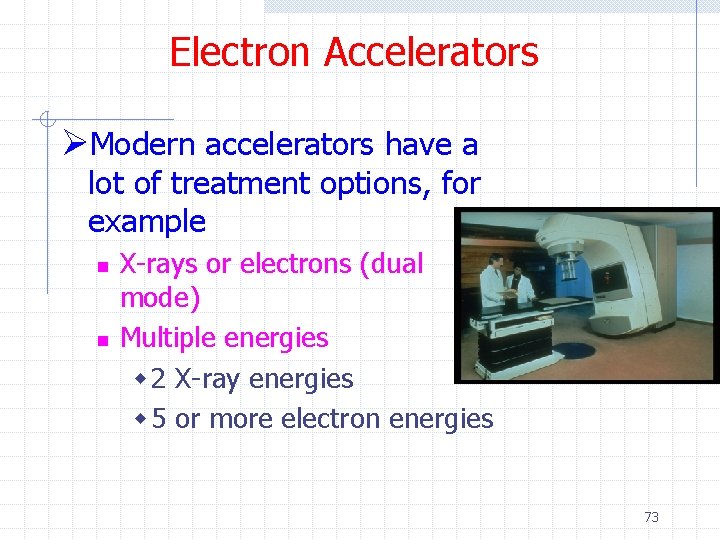
Electron Accelerators ØModern accelerators have a lot of treatment options, for example n n X-rays or electrons (dual mode) Multiple energies w 2 X-ray energies w 5 or more electron energies 73
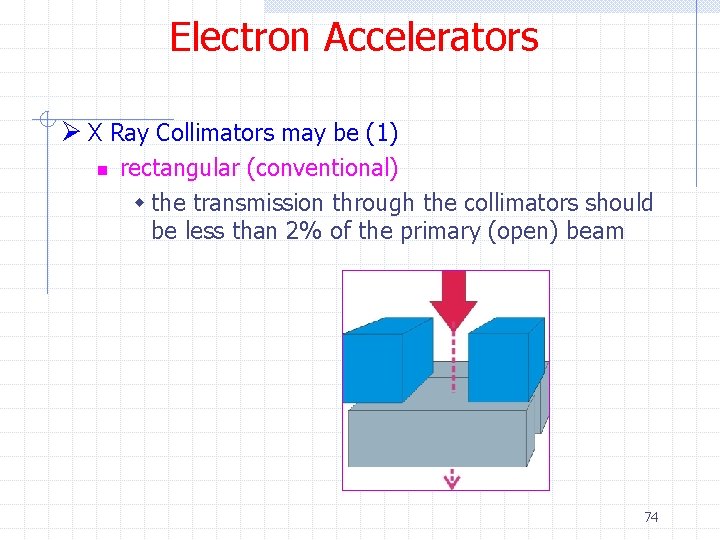
Electron Accelerators Ø X Ray Collimators may be (1) n rectangular (conventional) w the transmission through the collimators should be less than 2% of the primary (open) beam 74
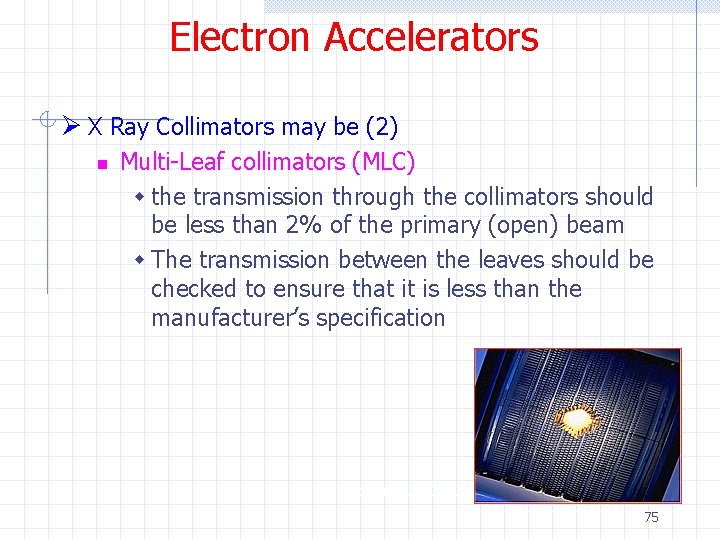
Electron Accelerators Ø X Ray Collimators may be (2) n Multi-Leaf collimators (MLC) w the transmission through the collimators should be less than 2% of the primary (open) beam w The transmission between the leaves should be checked to ensure that it is less than the manufacturer’s specification Siemens MLC 75
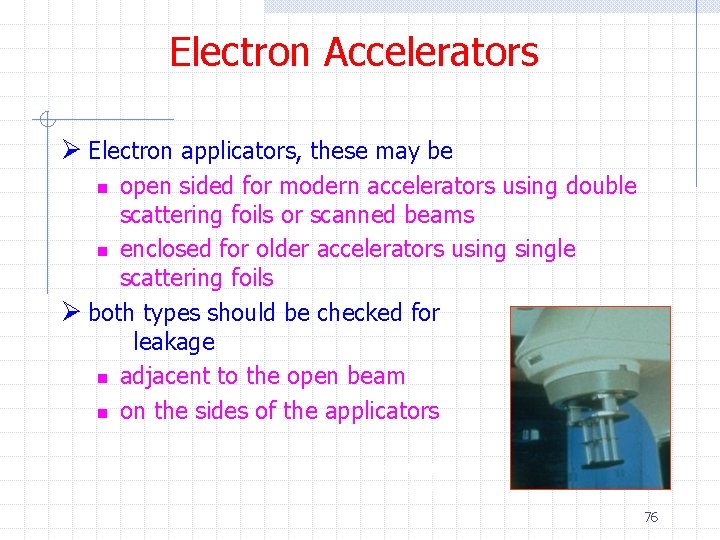
Electron Accelerators Ø Electron applicators, these may be open sided for modern accelerators using double scattering foils or scanned beams n enclosed for older accelerators usingle scattering foils Ø both types should be checked for leakage n adjacent to the open beam n on the sides of the applicators n Varian open sided electron cone 76
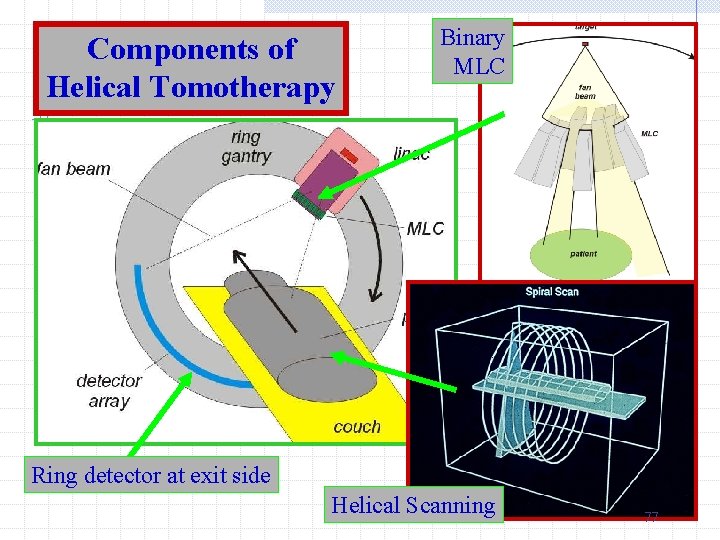
Components of Helical Tomotherapy Binary MLC Ring detector at exit side Helical Scanning 77
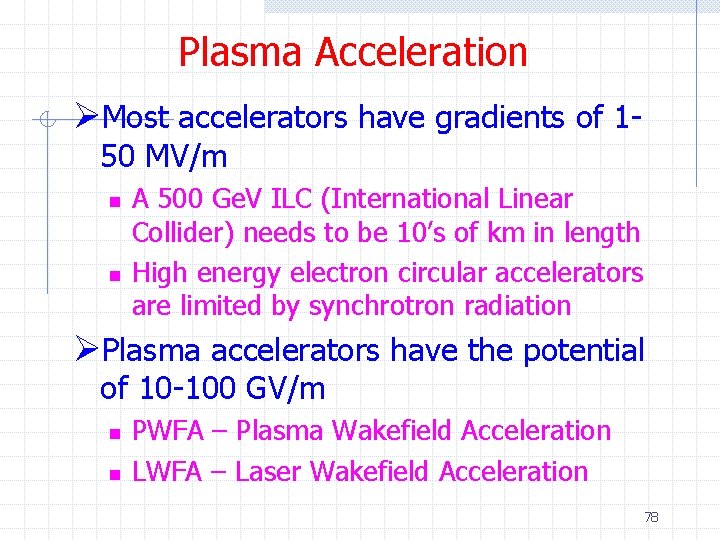
Plasma Acceleration ØMost accelerators have gradients of 150 MV/m n n A 500 Ge. V ILC (International Linear Collider) needs to be 10’s of km in length High energy electron circular accelerators are limited by synchrotron radiation ØPlasma accelerators have the potential of 10 -100 GV/m n n PWFA – Plasma Wakefield Acceleration LWFA – Laser Wakefield Acceleration 78
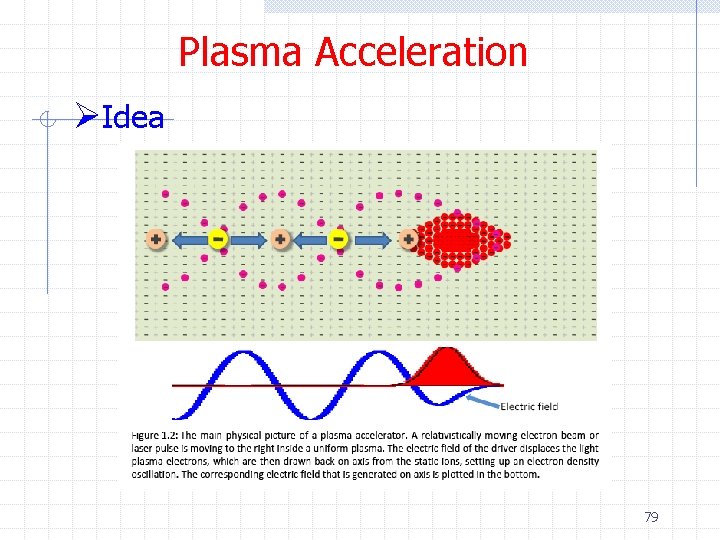
Plasma Acceleration ØIdea 79
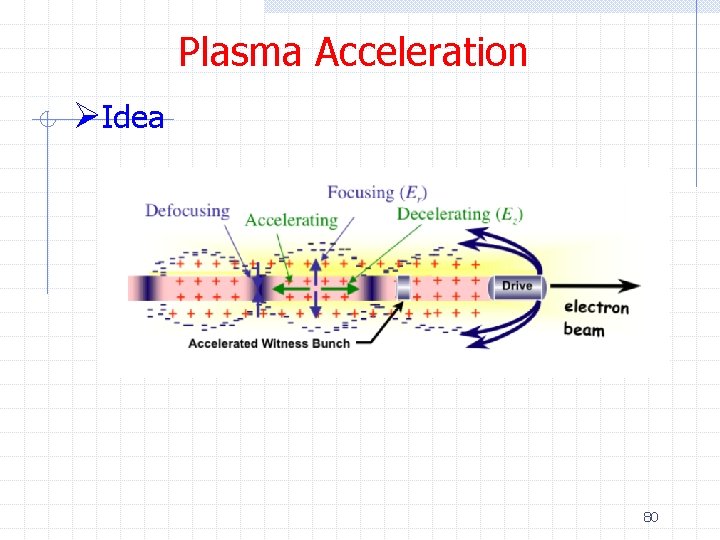
Plasma Acceleration ØIdea 80
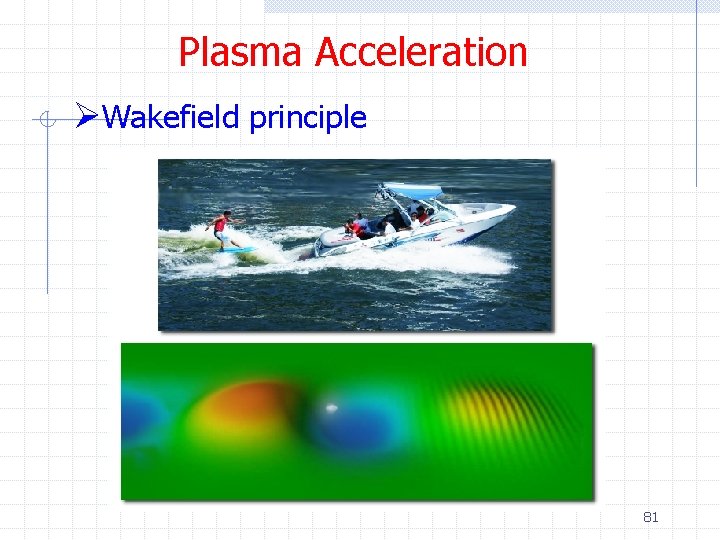
Plasma Acceleration ØWakefield principle 81
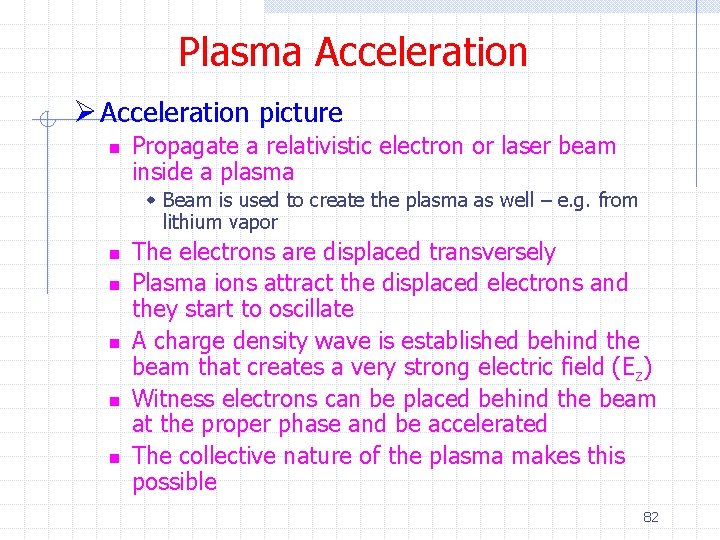
Plasma Acceleration Ø Acceleration picture n Propagate a relativistic electron or laser beam inside a plasma w Beam is used to create the plasma as well – e. g. from lithium vapor n n n The electrons are displaced transversely Plasma ions attract the displaced electrons and they start to oscillate A charge density wave is established behind the beam that creates a very strong electric field (Ez) Witness electrons can be placed behind the beam at the proper phase and be accelerated The collective nature of the plasma makes this possible 82
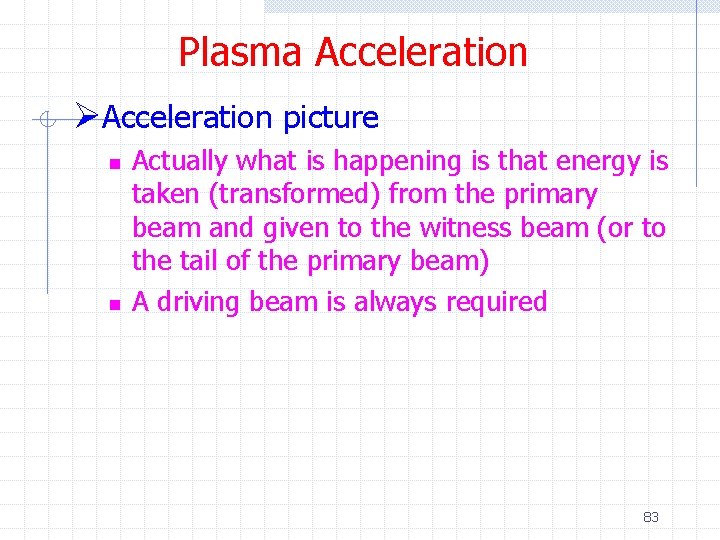
Plasma Acceleration ØAcceleration picture n n Actually what is happening is that energy is taken (transformed) from the primary beam and given to the witness beam (or to the tail of the primary beam) A driving beam is always required 83
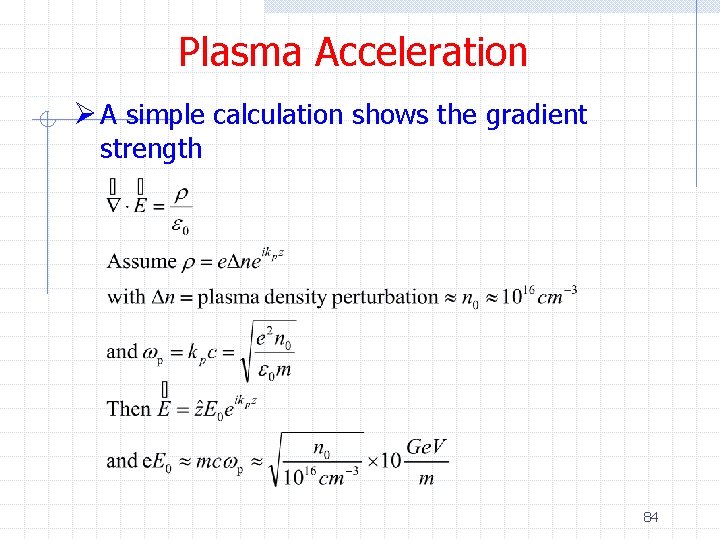
Plasma Acceleration Ø A simple calculation shows the gradient strength 84
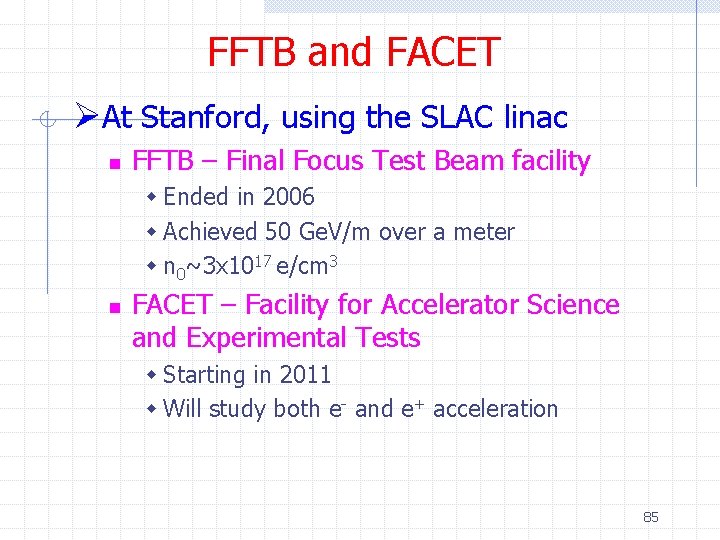
FFTB and FACET ØAt Stanford, using the SLAC linac n FFTB – Final Focus Test Beam facility w Ended in 2006 w Achieved 50 Ge. V/m over a meter w n 0~3 x 1017 e/cm 3 n FACET – Facility for Accelerator Science and Experimental Tests w Starting in 2011 w Will study both e- and e+ acceleration 85
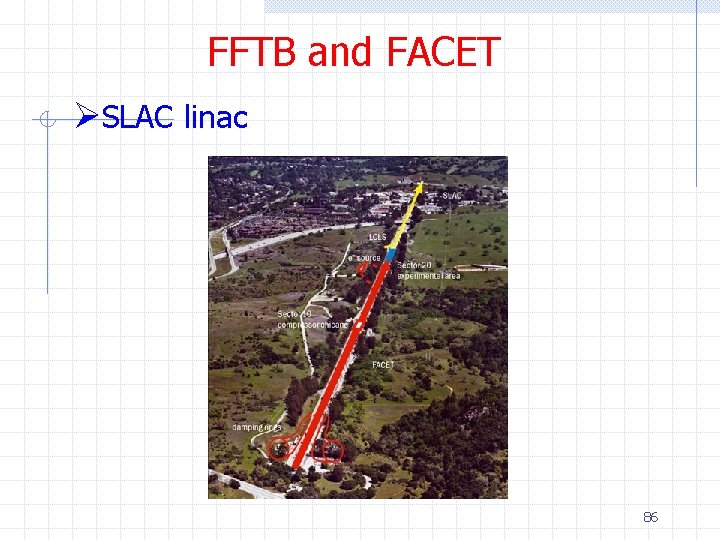
FFTB and FACET ØSLAC linac 86
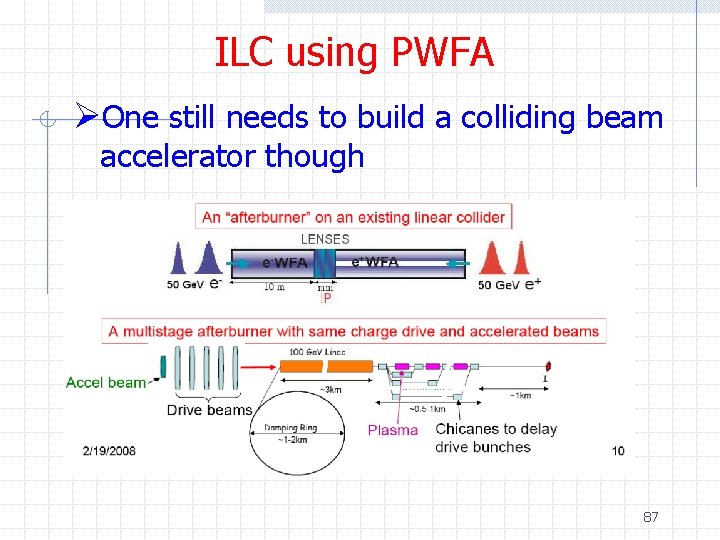
ILC using PWFA ØOne still needs to build a colliding beam accelerator though 87
Cyclotron and synchrotron radiation
X.next = x.next.next
After me after me after me
If any man come after me
Formula of cyclotron
Cyclotron
Cyclotron
Cyclotron diagram
Rozi
Antaya science and technology
Baby cyclotron
Synchrotron radiation
National synchrotron light source ii
Photon density formula
Lenny rivkin
Synchrotron radiation
Psi synchrotron
Stanford
Synchrotron
Synchrotron
Felexit
Synchrotron radiation
Synchrotron
Synchrotron
Synchrotron radiation
Synchrotron radiation
What is after the introduction
What's next thinking about life after high school
Example of main idea
What is controlling idea
Contoh penanda organisasi
Is central message and theme the same thing
Is the main idea the theme
Exegetical idea vs homiletical idea
A supporting sentence
đặc điểm cơ thể của người tối cổ
Vẽ hình chiếu vuông góc của vật thể sau
Các châu lục và đại dương trên thế giới
Glasgow thang điểm
ưu thế lai là gì
Tư thế ngồi viết
Bàn tay mà dây bẩn
Các châu lục và đại dương trên thế giới
Mật thư anh em như thể tay chân
Bổ thể
Tư thế ngồi viết
Thế nào là giọng cùng tên?
Thẻ vin
Thể thơ truyền thống
Hát lên người ơi alleluia
Khi nào hổ con có thể sống độc lập
Từ ngữ thể hiện lòng nhân hậu
Diễn thế sinh thái là
Vẽ hình chiếu vuông góc của vật thể sau
101012 bằng
Tỉ lệ cơ thể trẻ em
Lời thề hippocrates
Vẽ hình chiếu đứng bằng cạnh của vật thể
đại từ thay thế
Quá trình desamine hóa có thể tạo ra
Môn thể thao bắt đầu bằng chữ f
Cong thức tính động năng
Hình ảnh bộ gõ cơ thể búng tay
Sự nuôi và dạy con của hươu
Thế nào là mạng điện lắp đặt kiểu nổi
Các loại đột biến cấu trúc nhiễm sắc thể
Nguyên nhân của sự mỏi cơ sinh 8
độ dài liên kết
Chó sói
Thiếu nhi thế giới liên hoan
điện thế nghỉ
Một số thể thơ truyền thống
Thế nào là hệ số cao nhất
Trời xanh đây là của chúng ta thể thơ
Sơ đồ cơ thể người
So nguyen to
Next hfa 115
Next bew moon
Abacus next
Special value testing
Next door by kurt vonnegut summary
Electrical energy
That man who lives on the next
Nclex next generation
Vendor management matrix
Pr pearsonaccessnext
Ellos ____ de perú