Chapter 5 The Working Cell Power Point Lectures
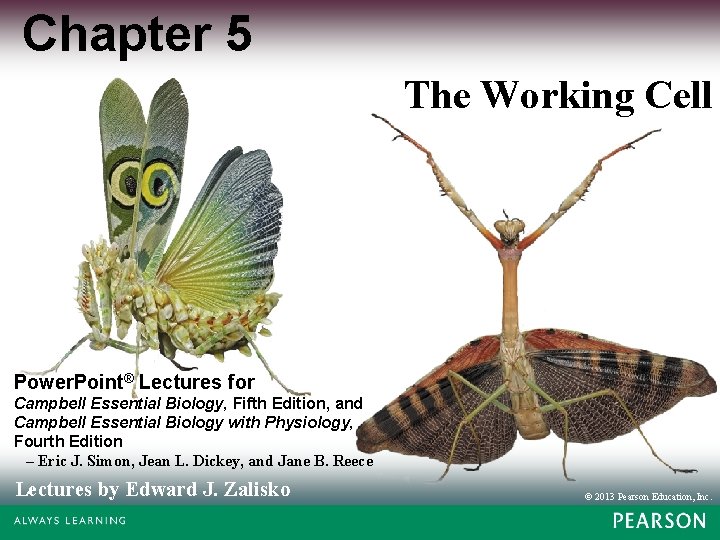
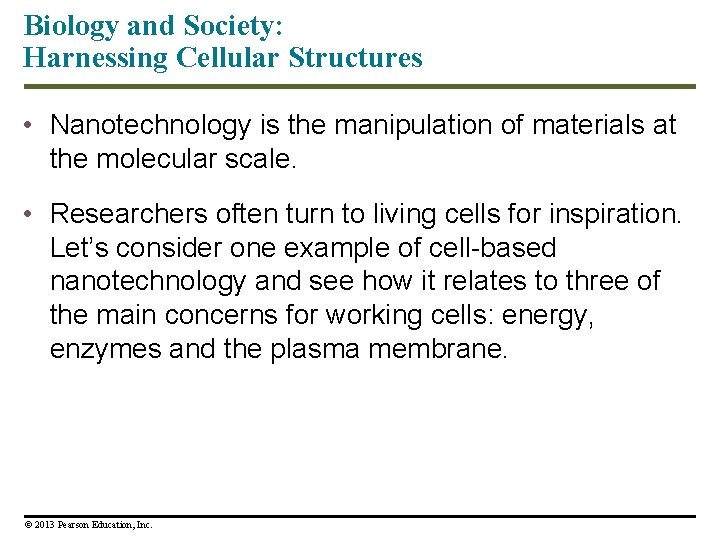
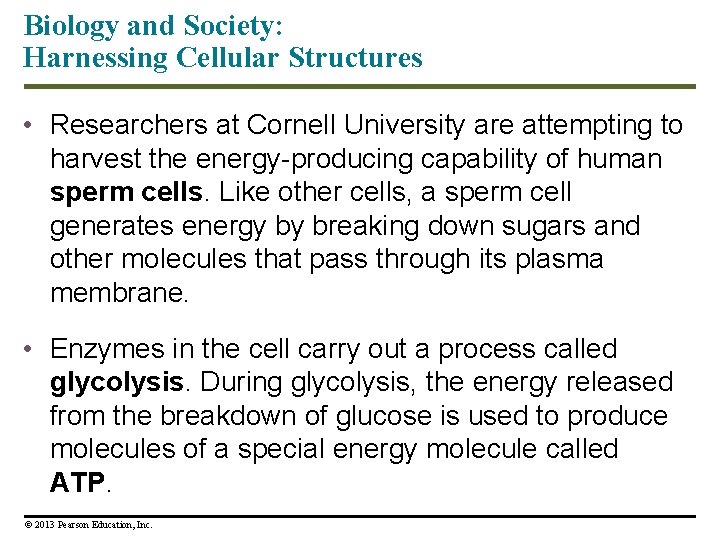
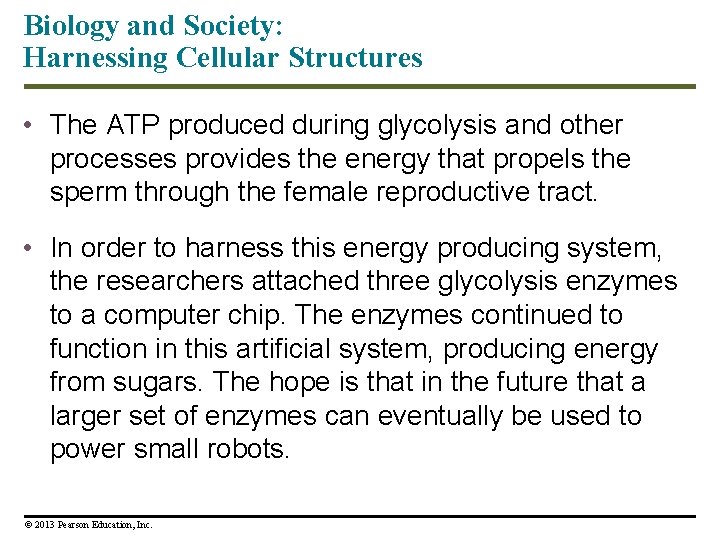
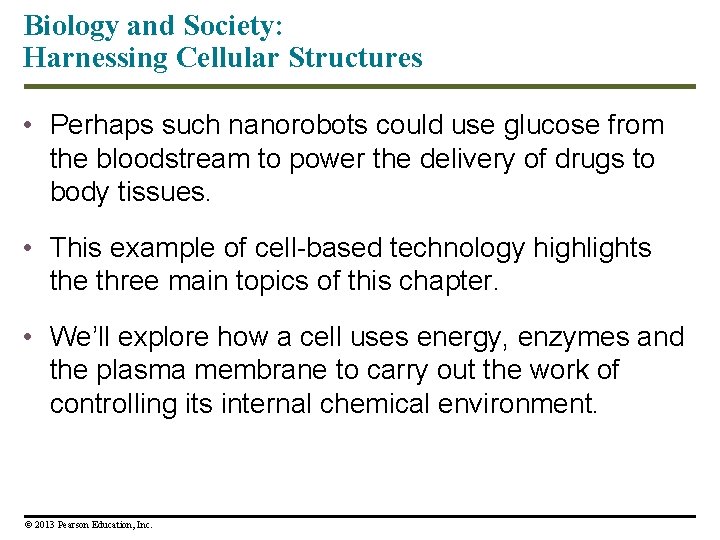
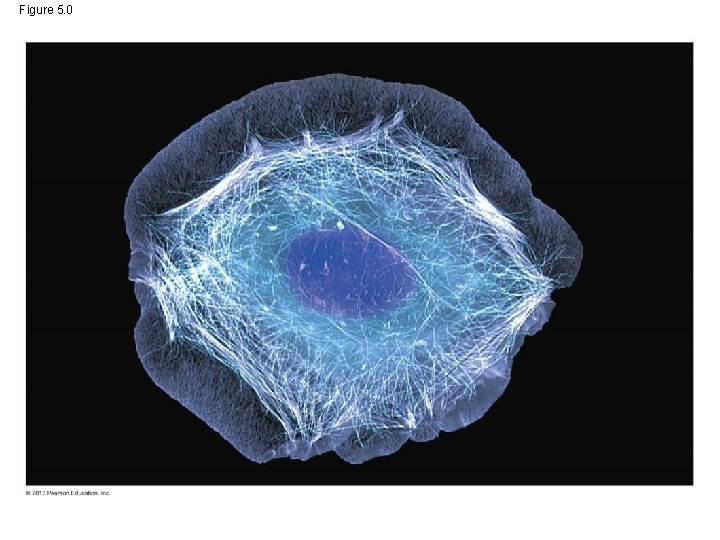
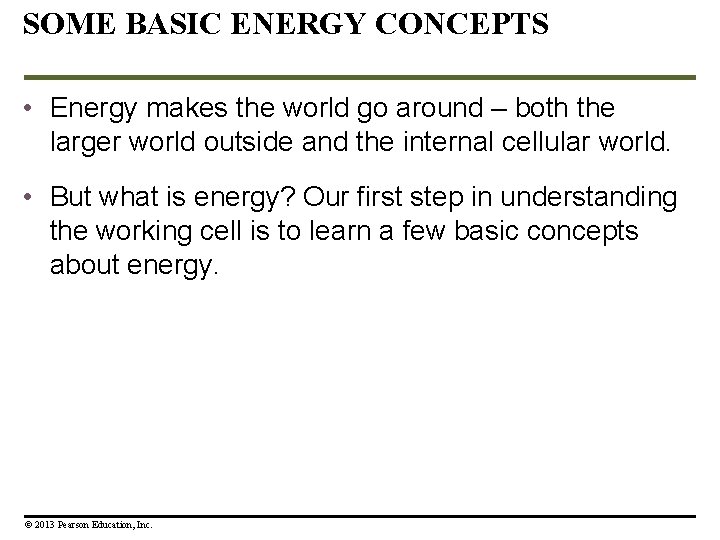
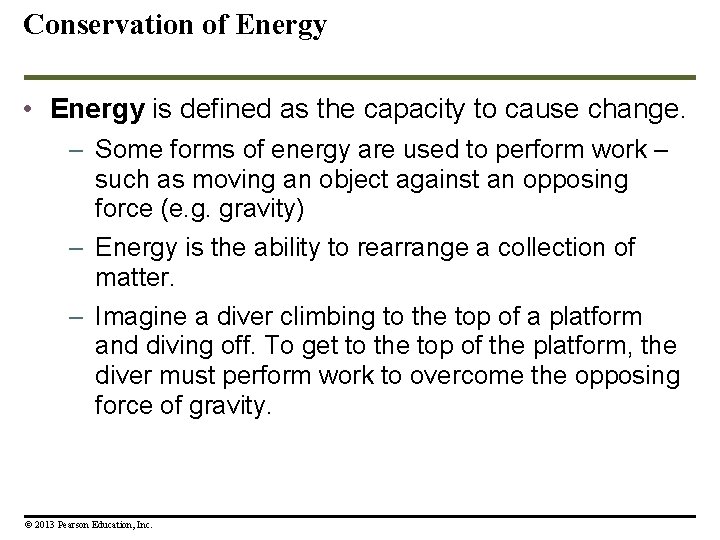
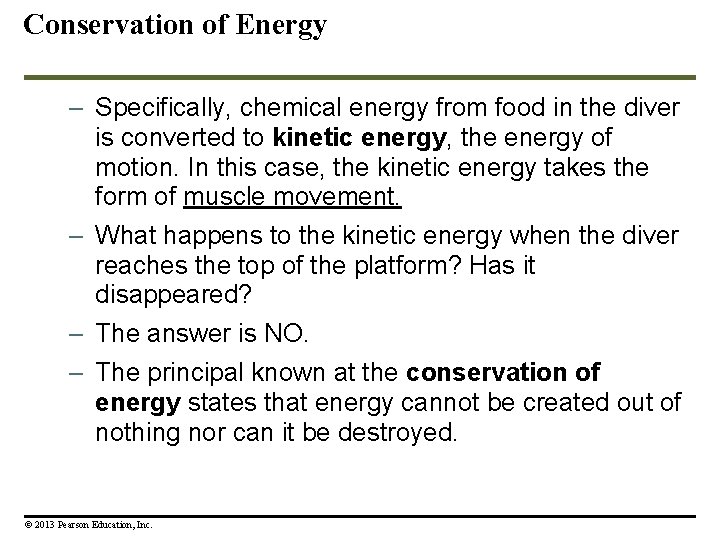
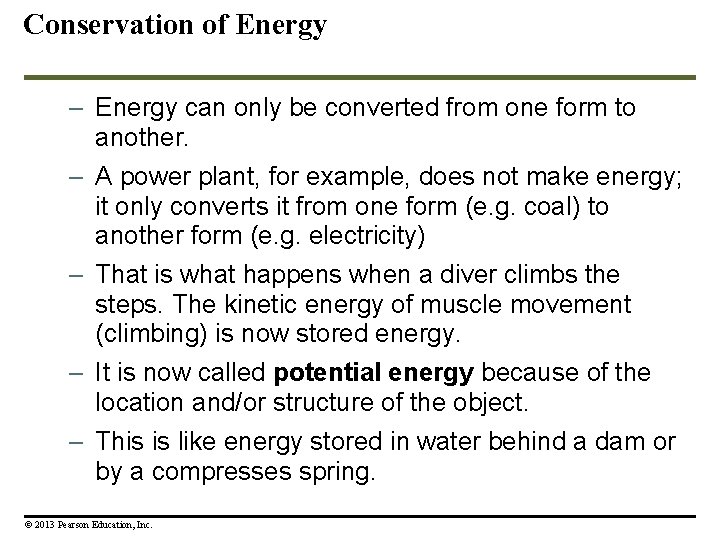
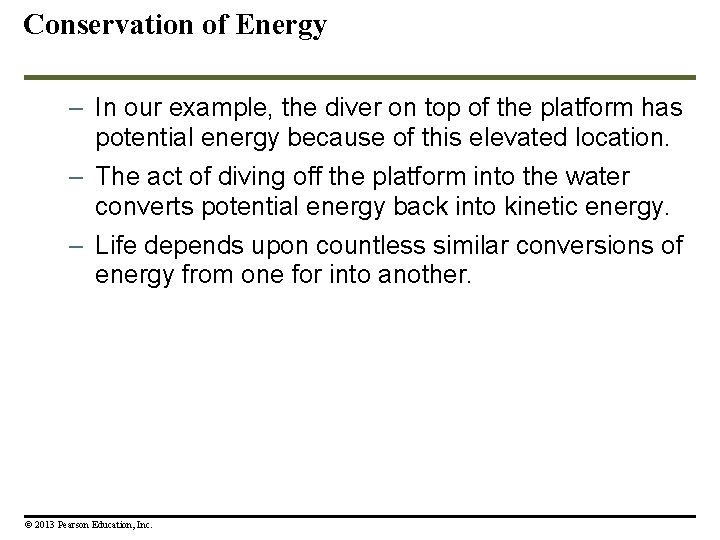
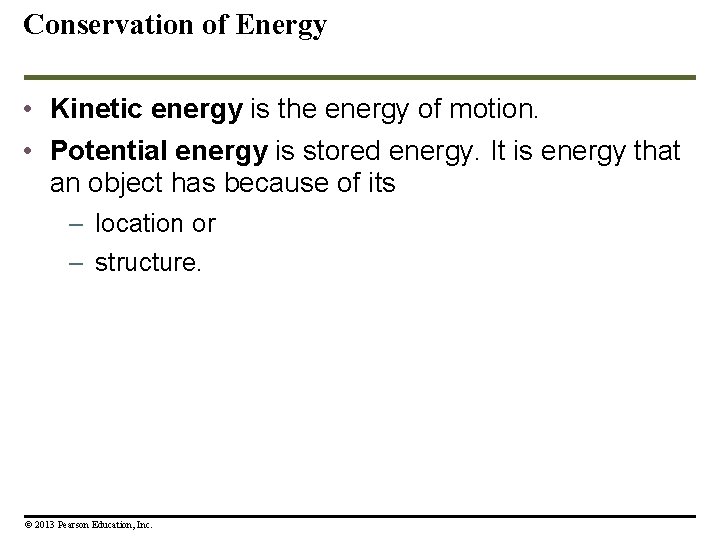
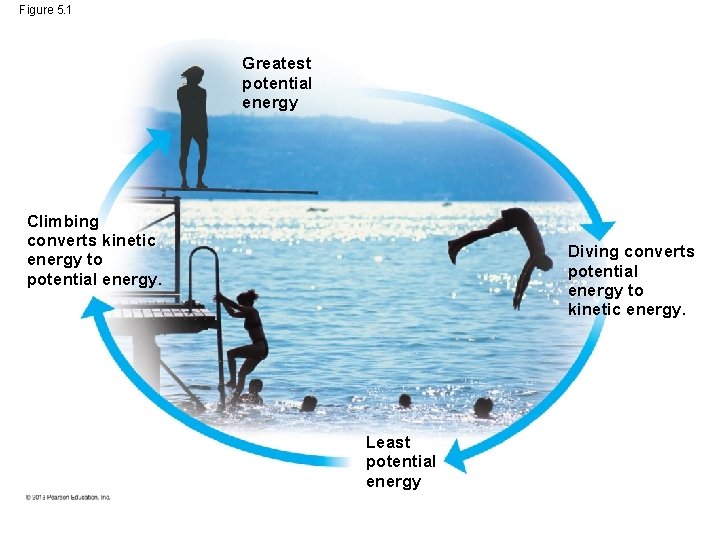
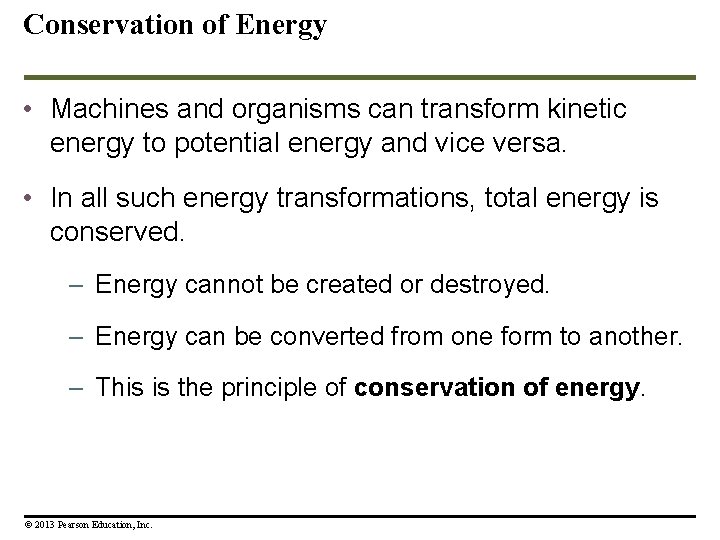
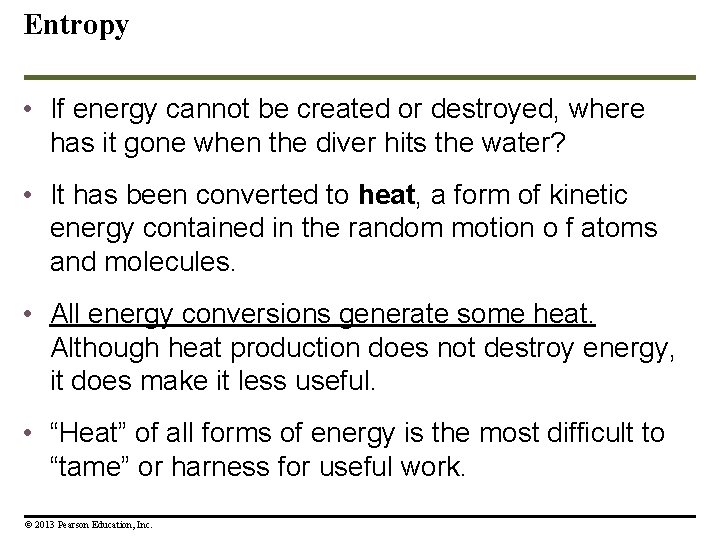
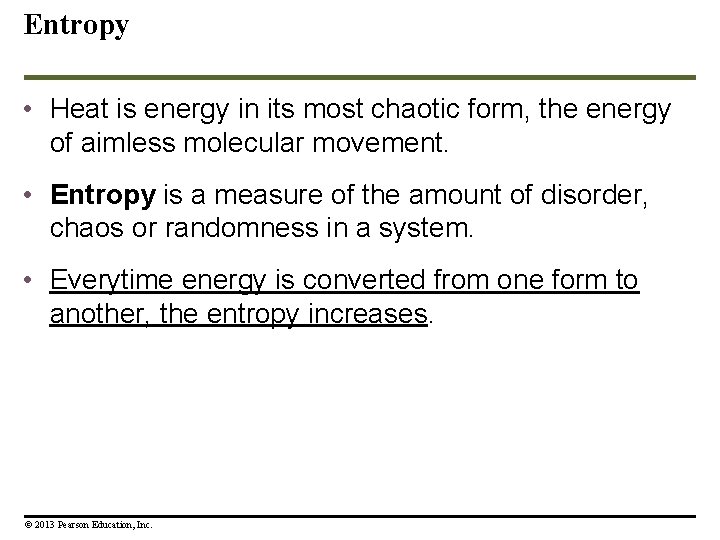
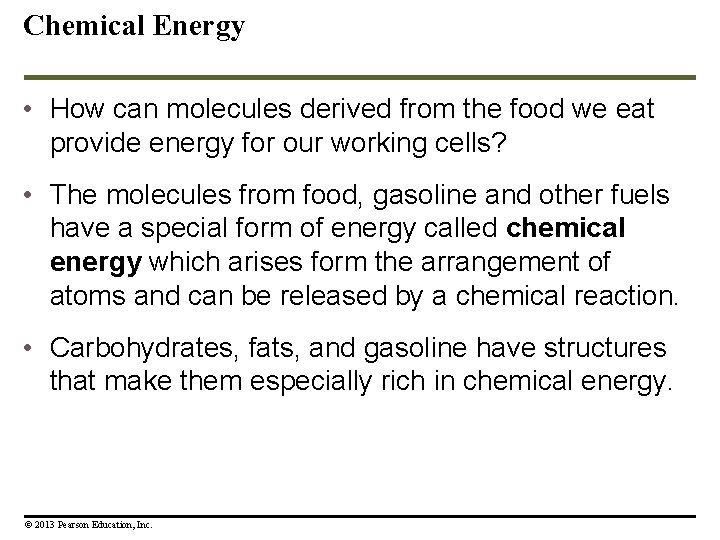
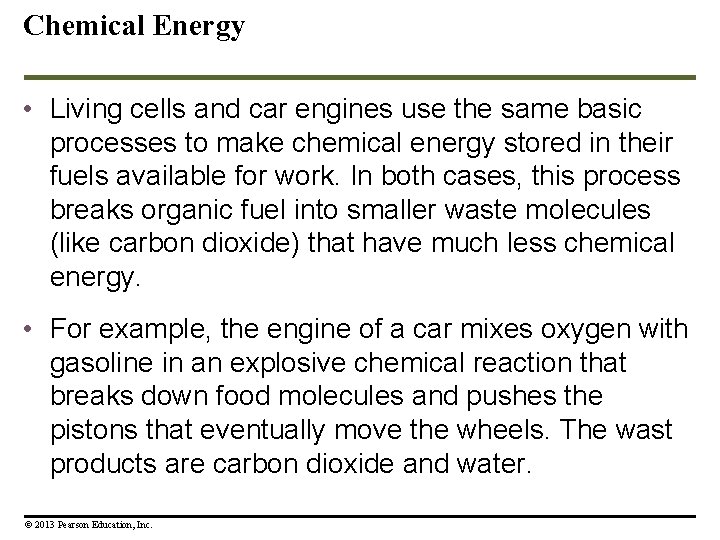
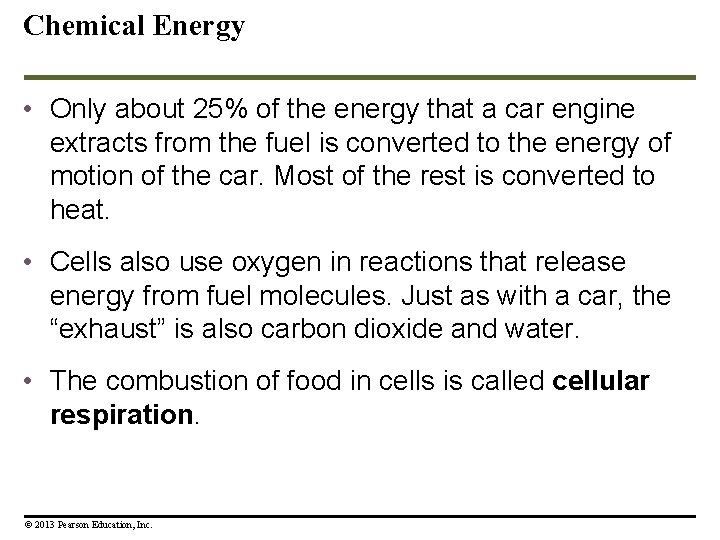
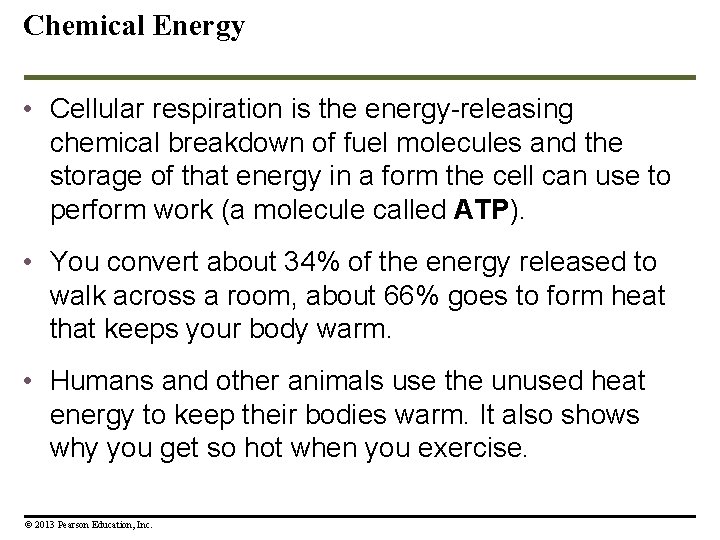
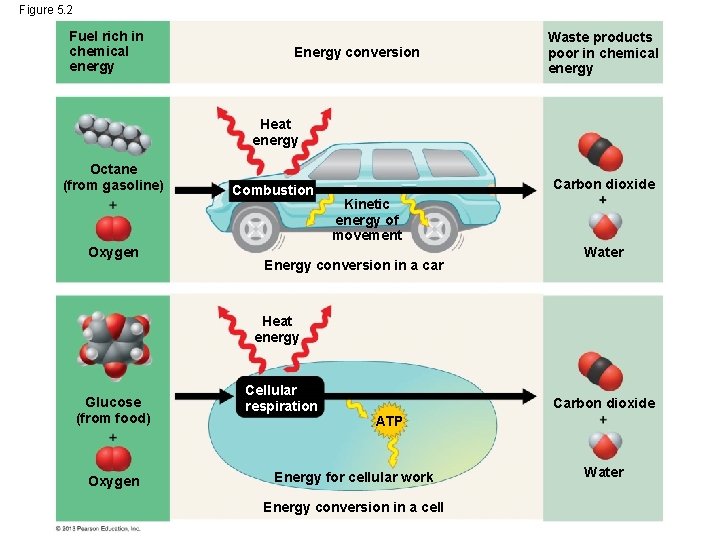
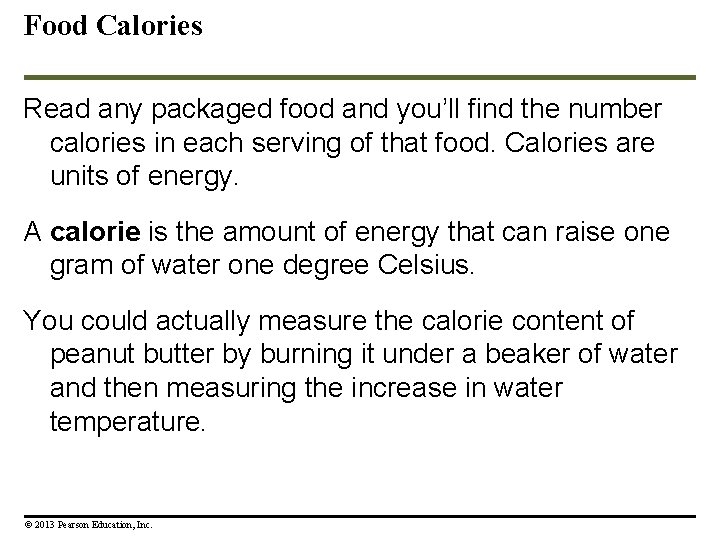
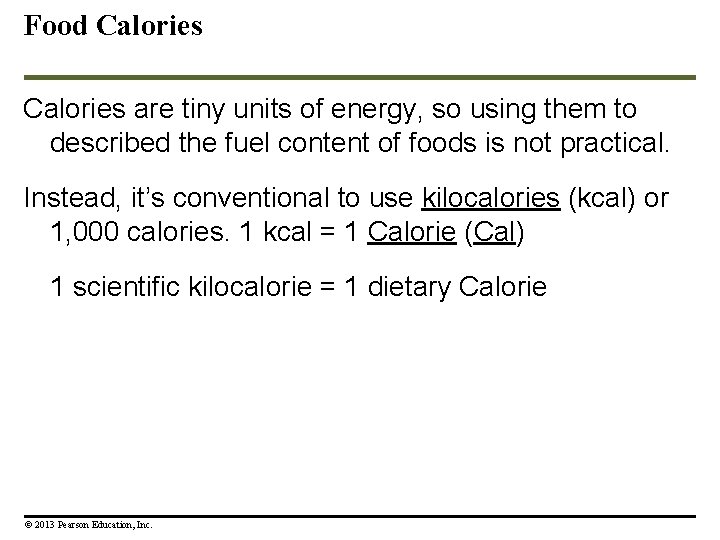
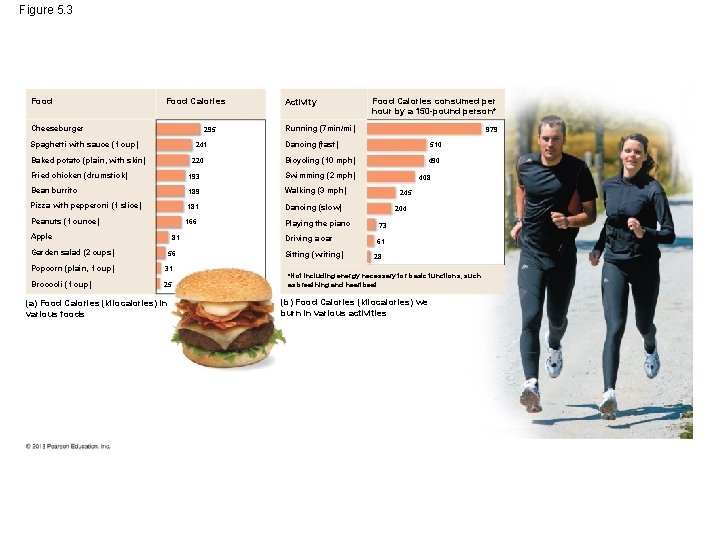
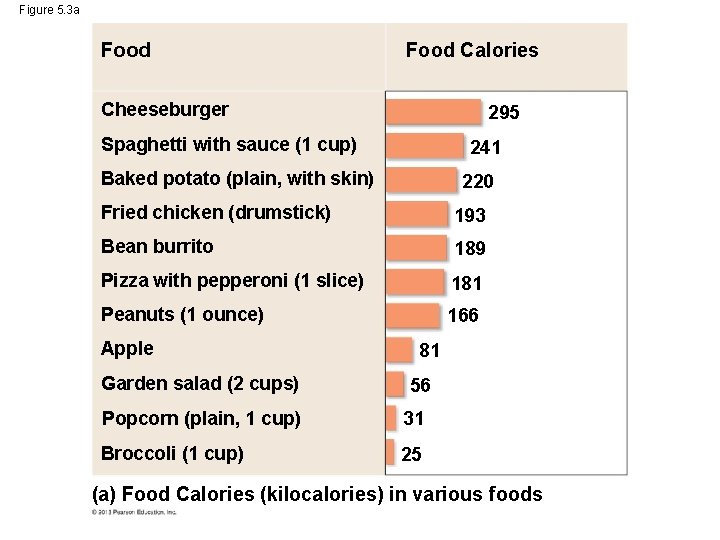
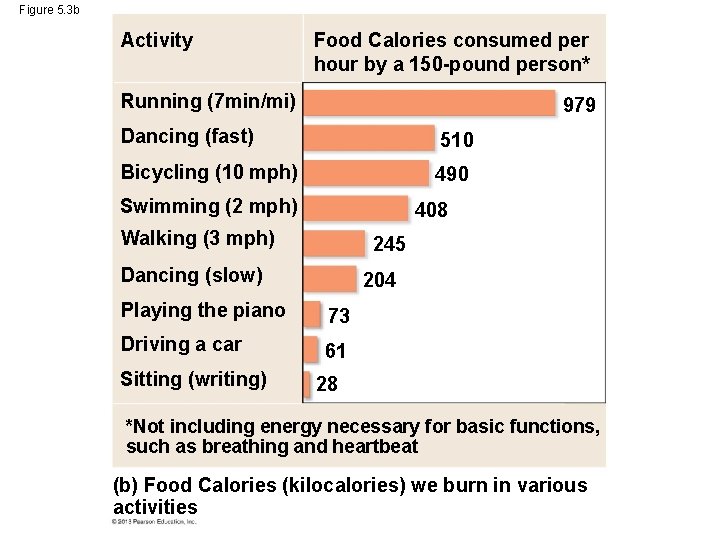
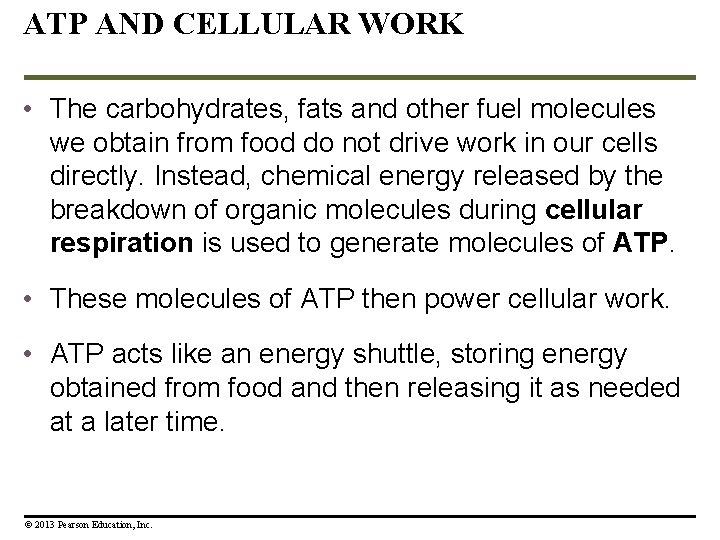
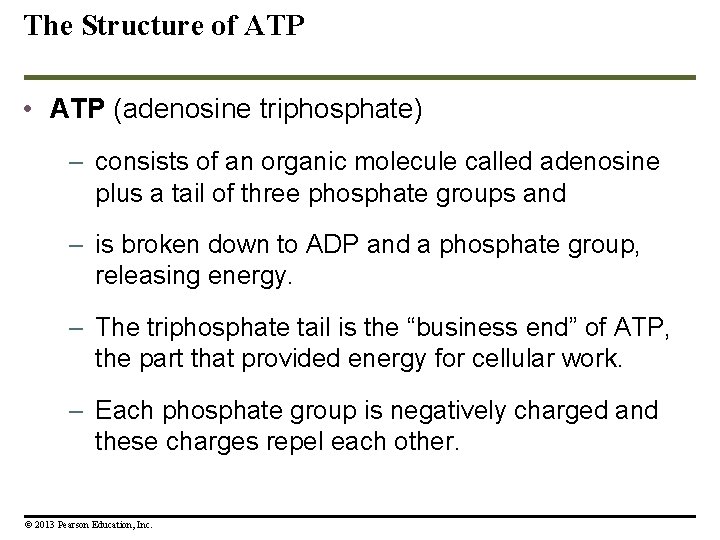
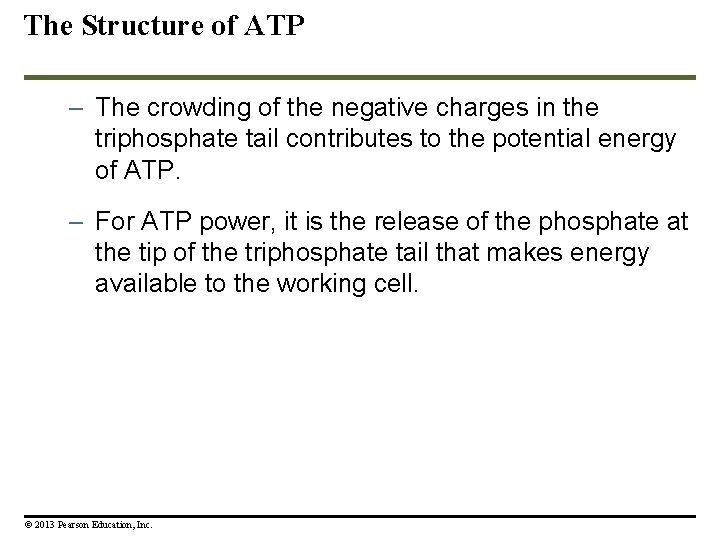
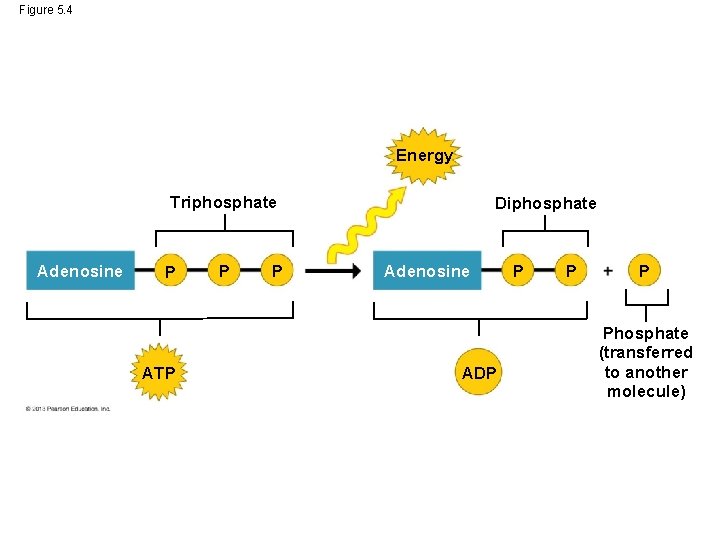
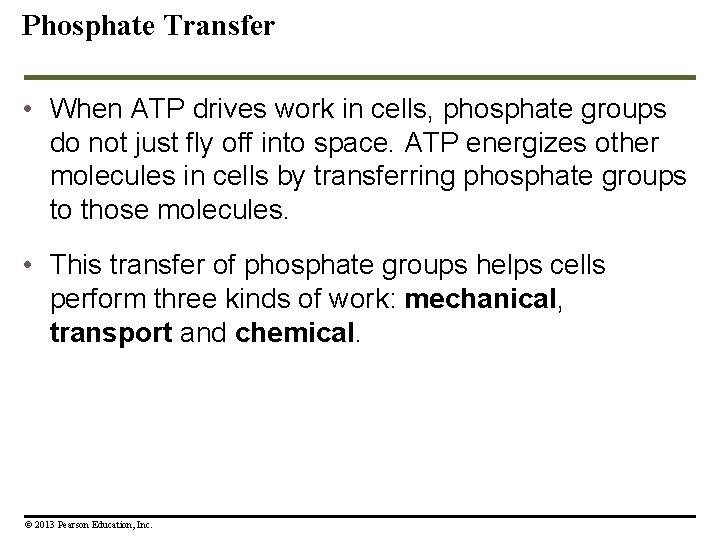
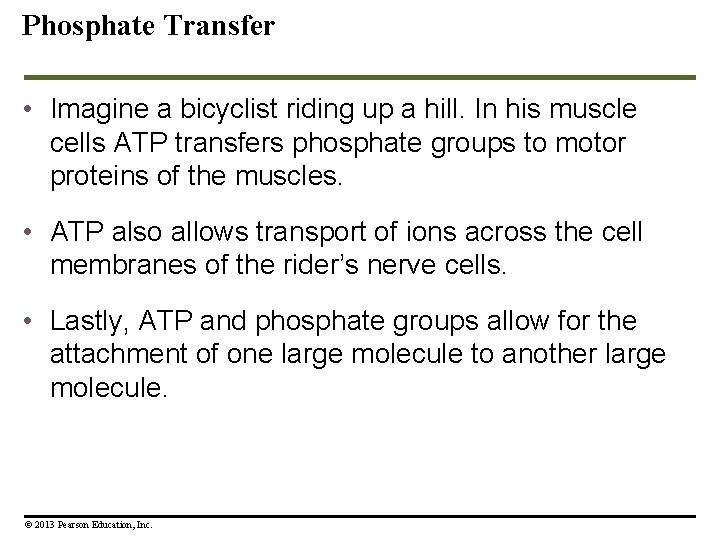
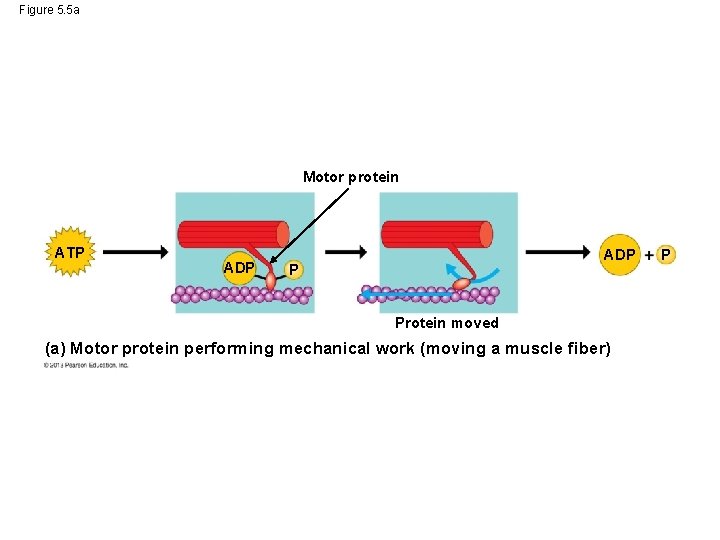
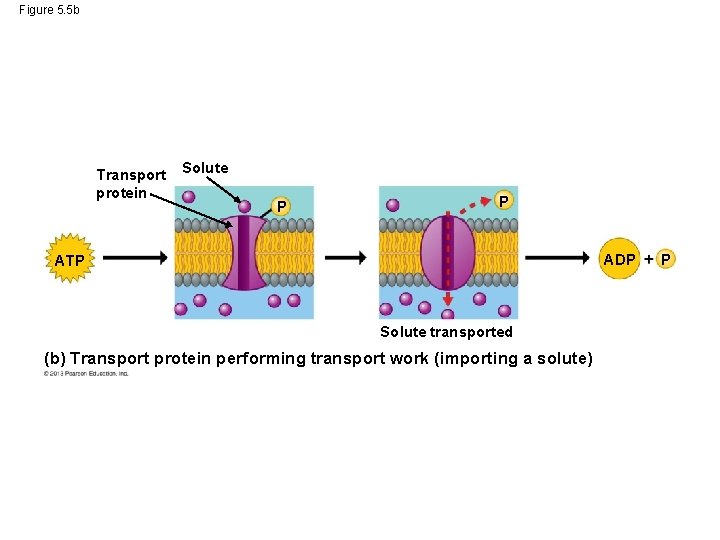
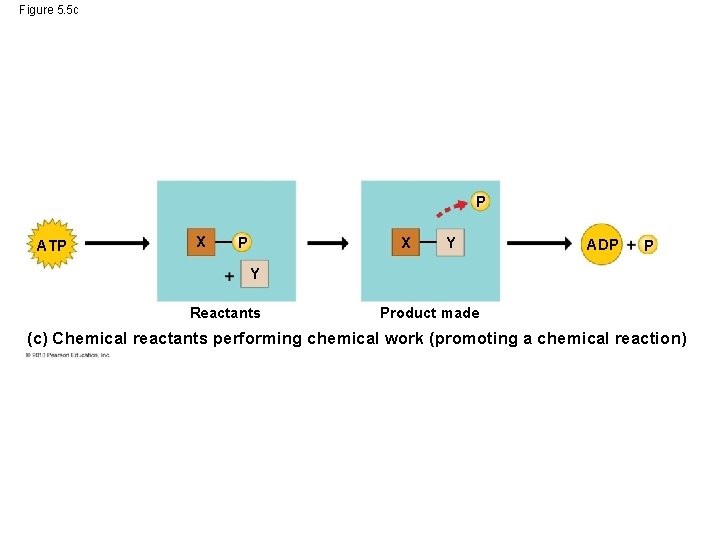
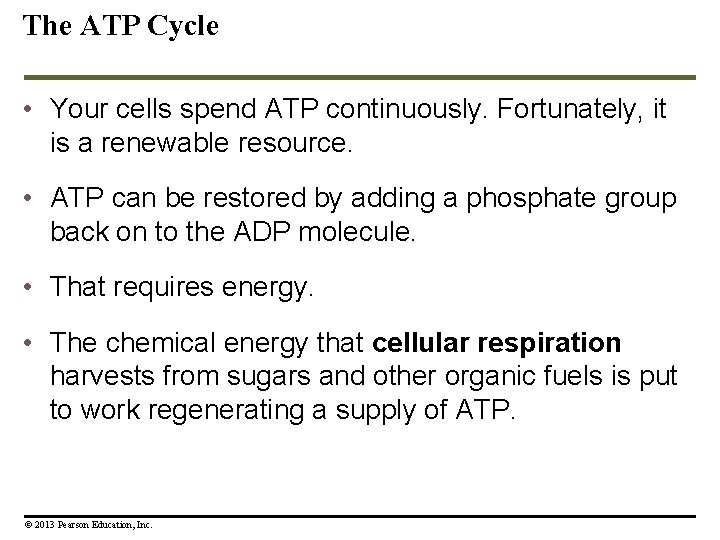
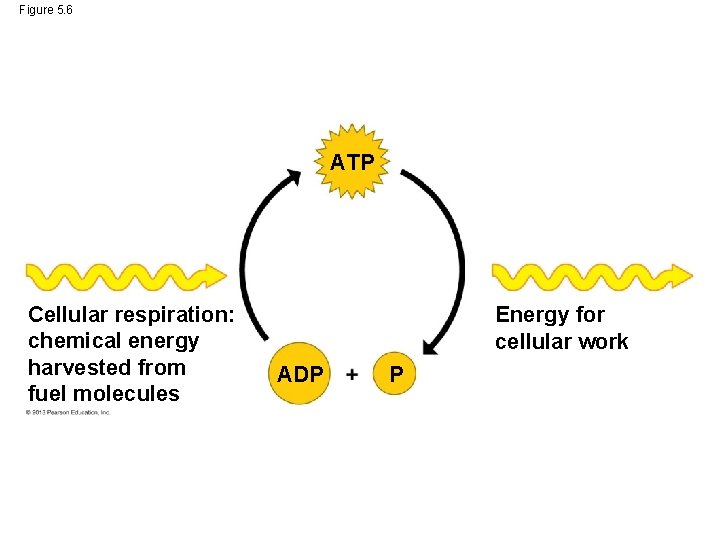
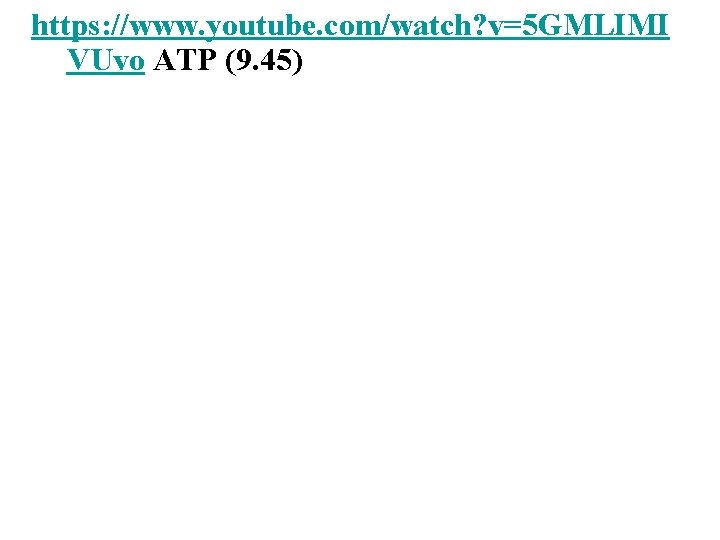
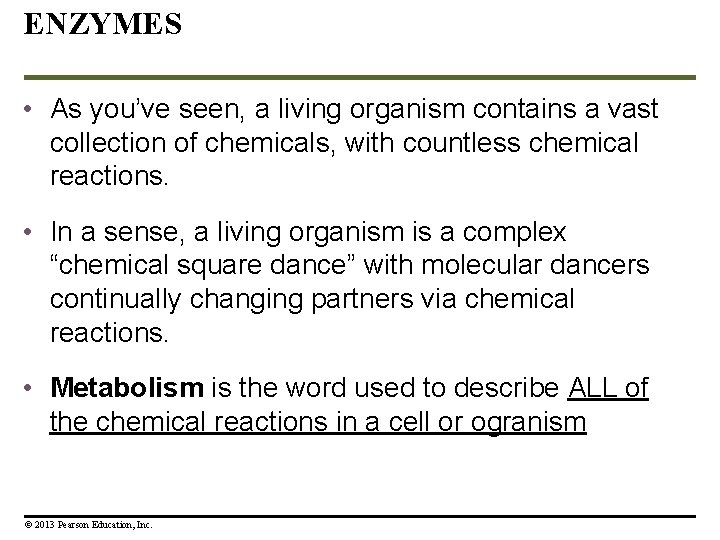
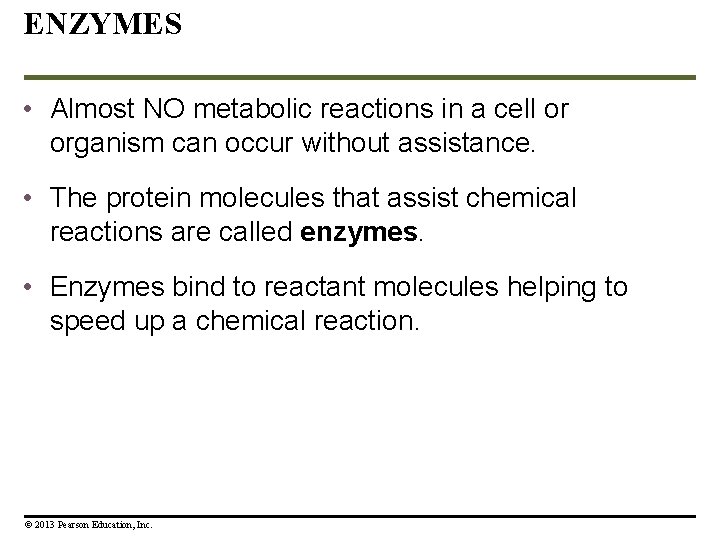
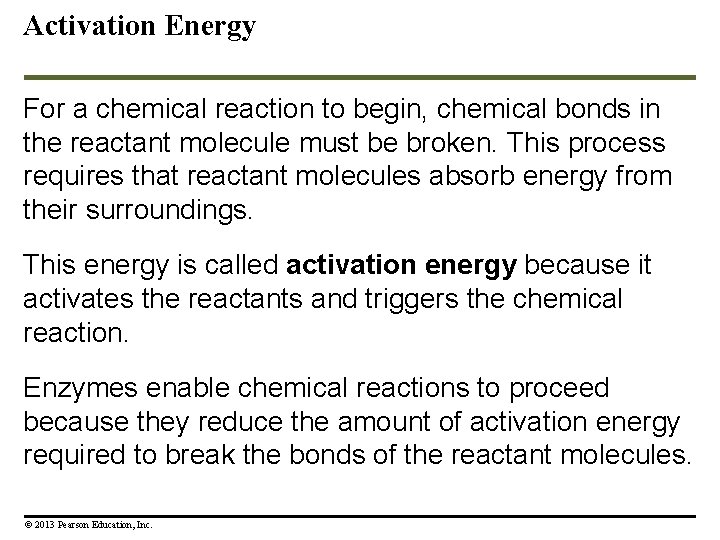
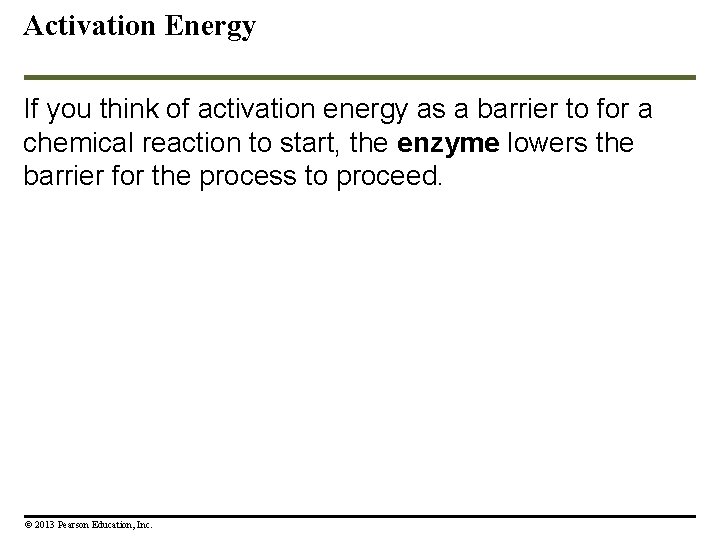
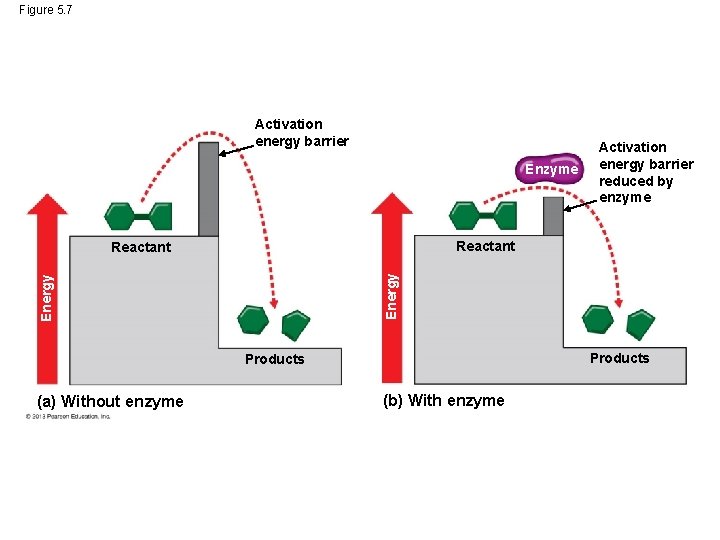
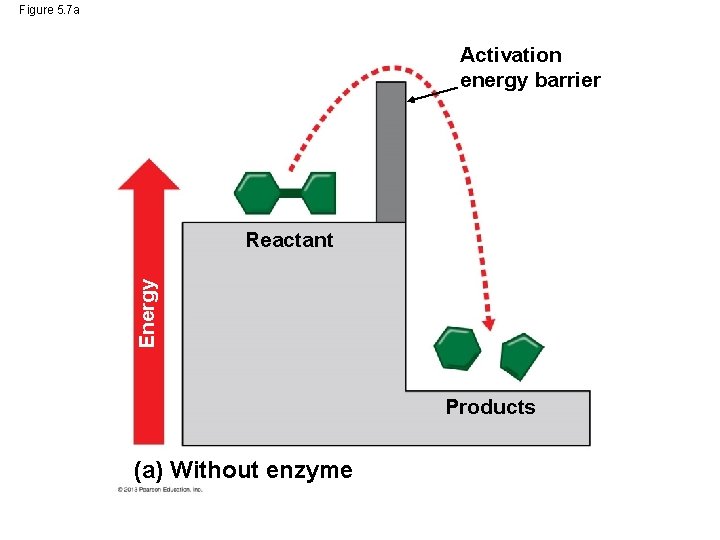
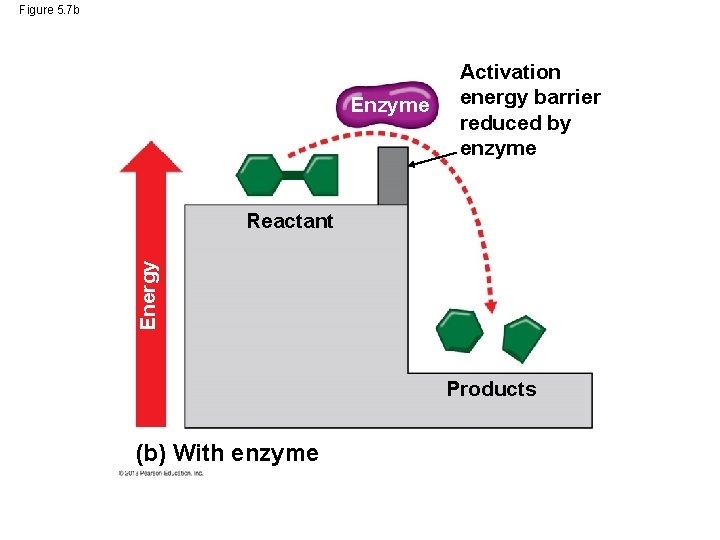
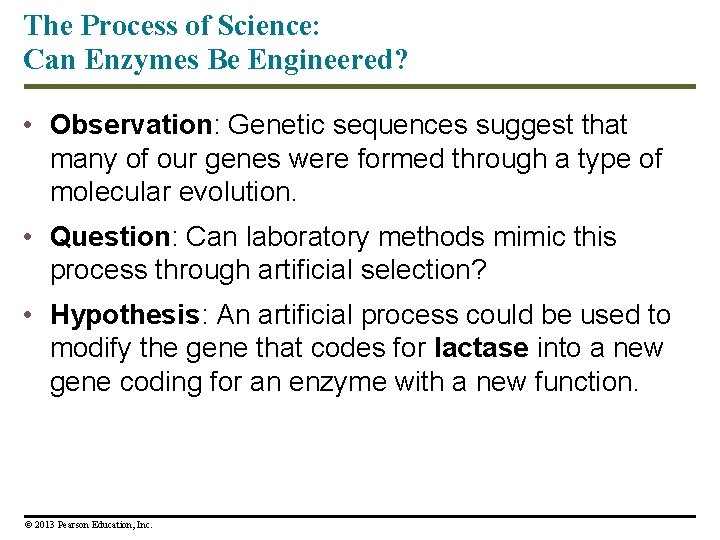
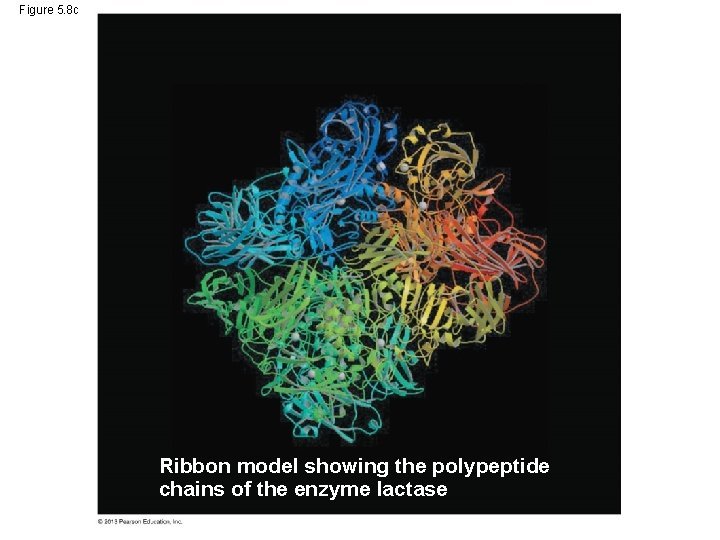
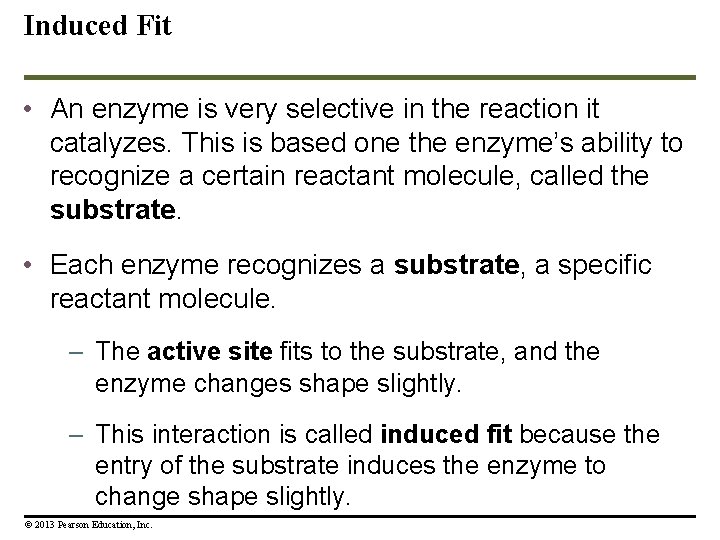
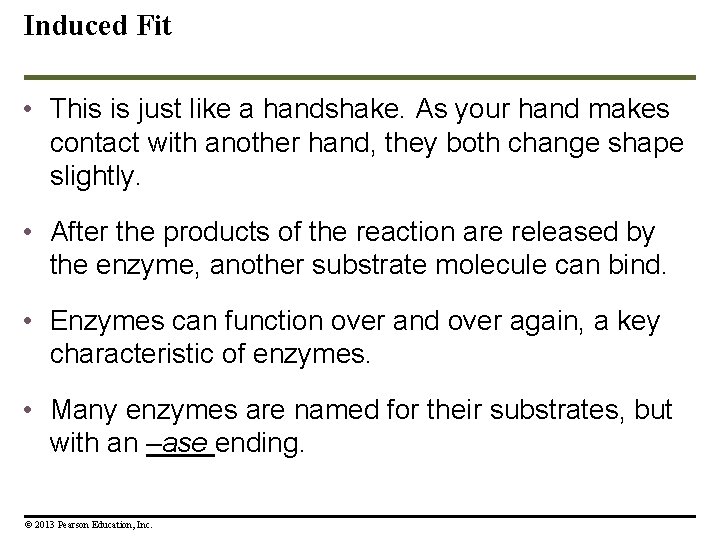
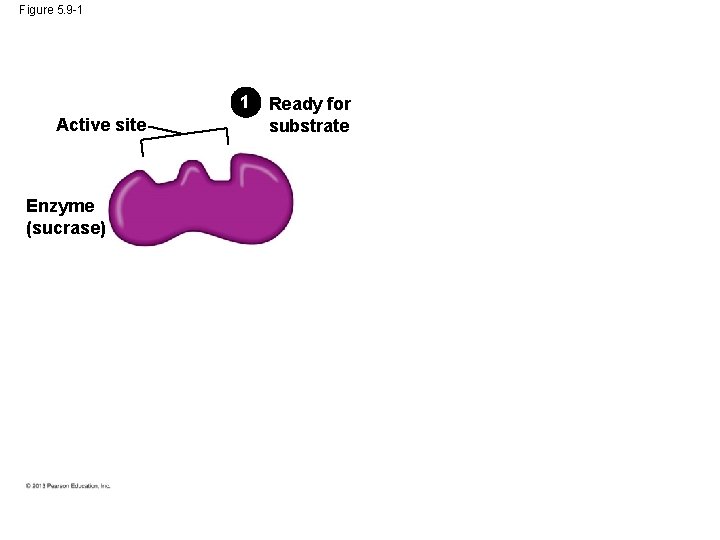
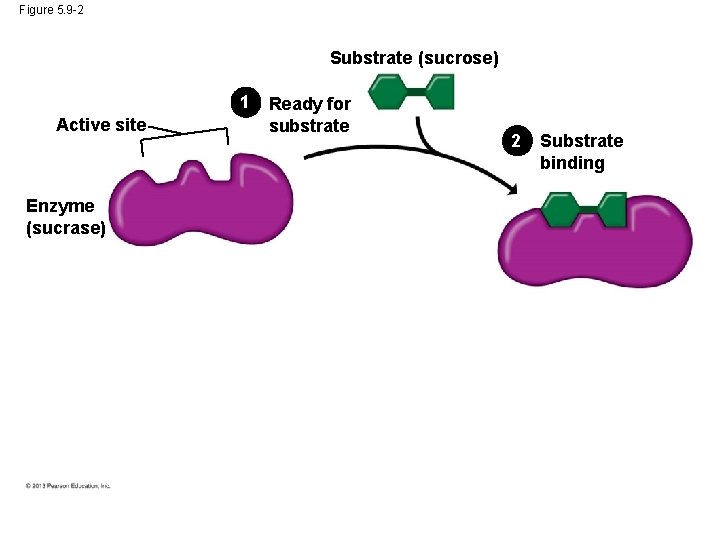
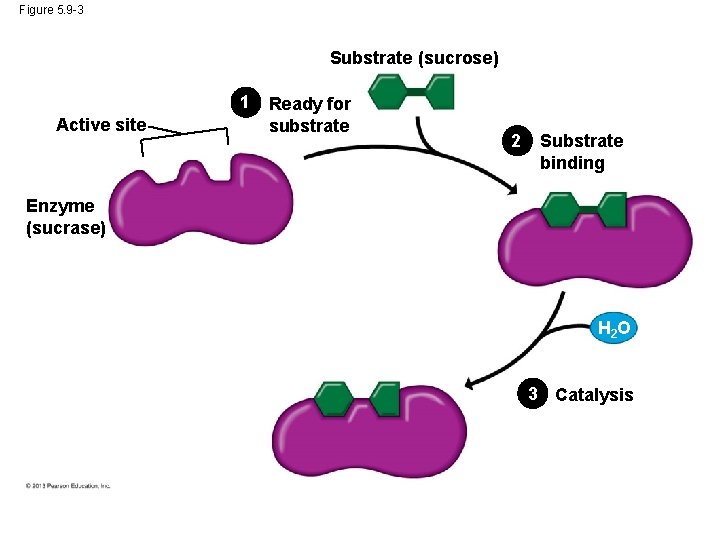
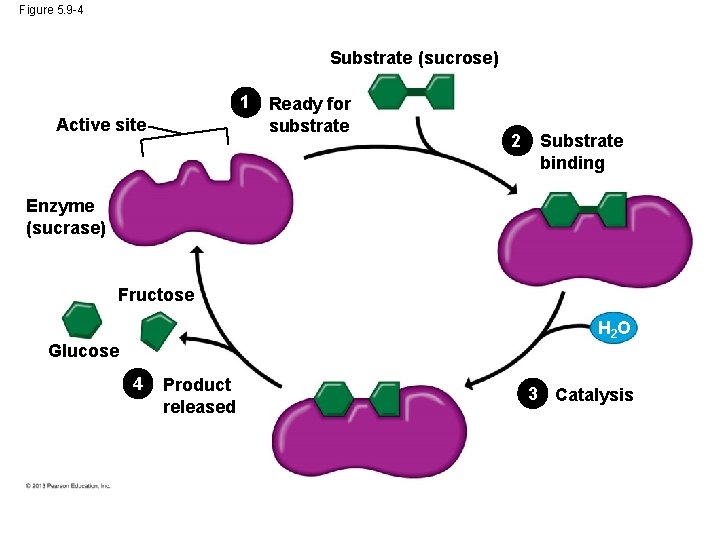
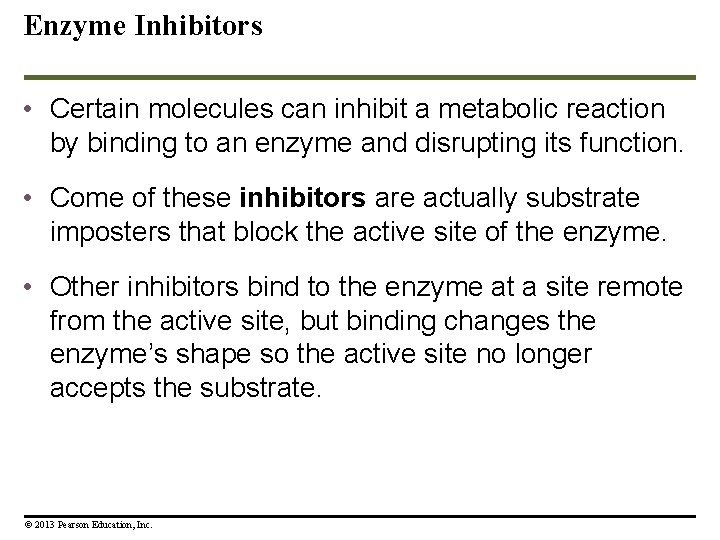
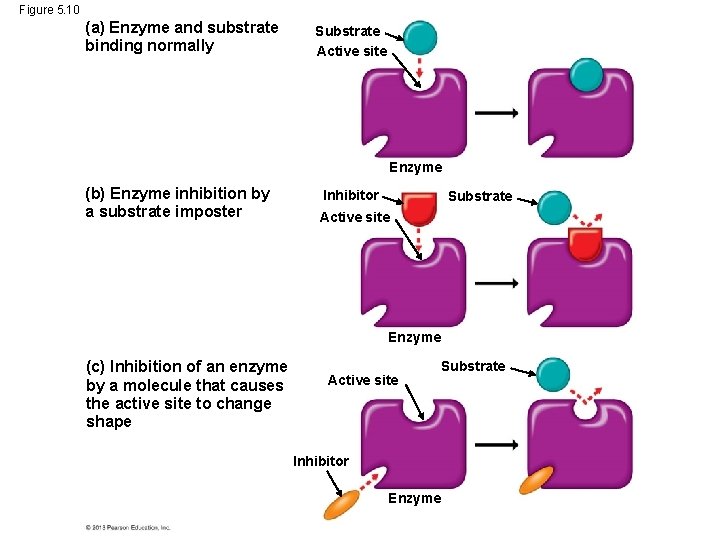
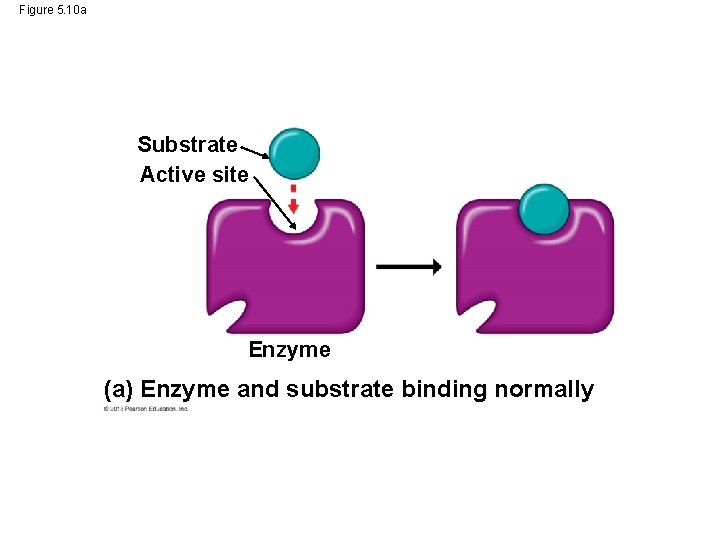
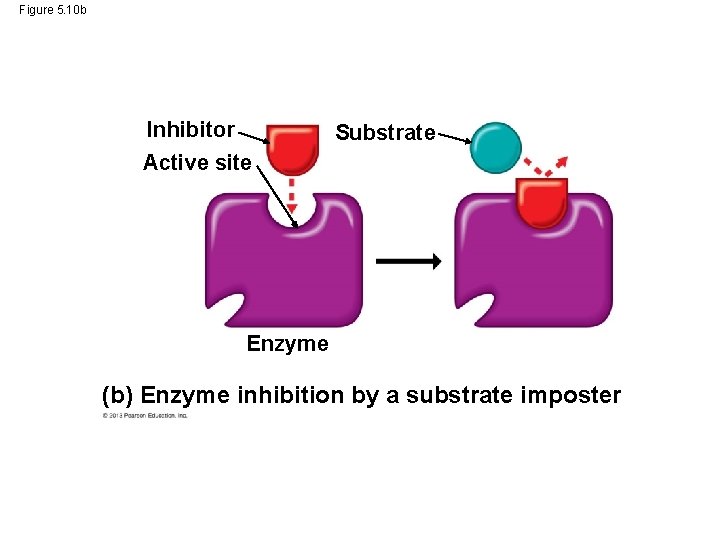
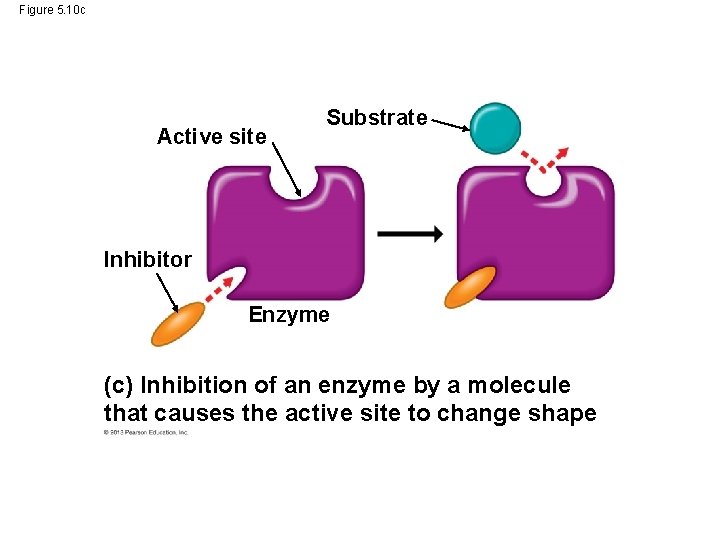
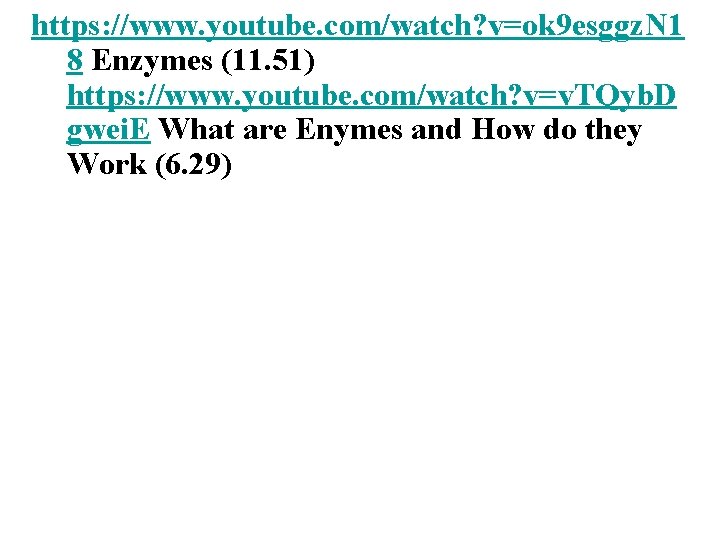
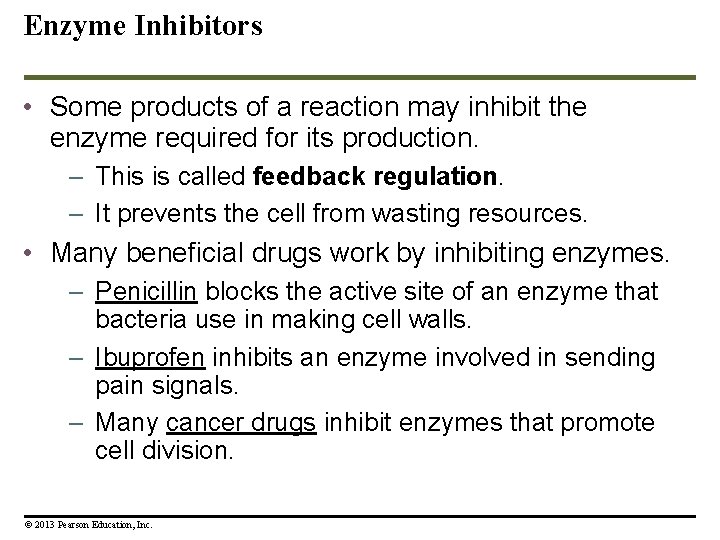
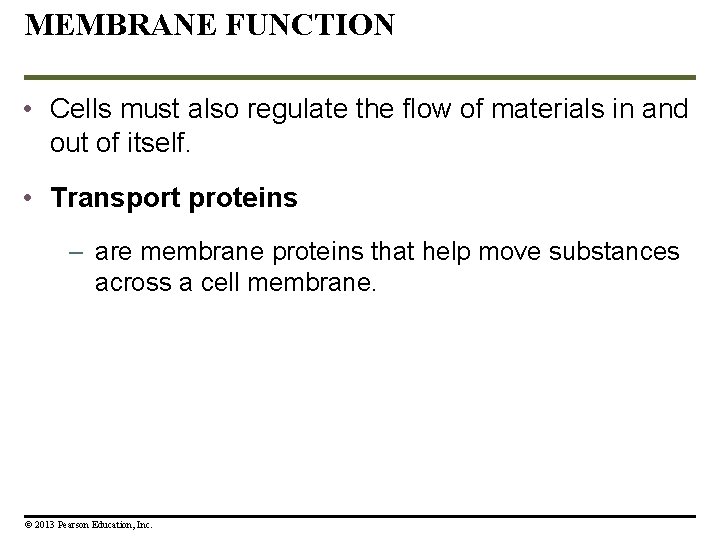
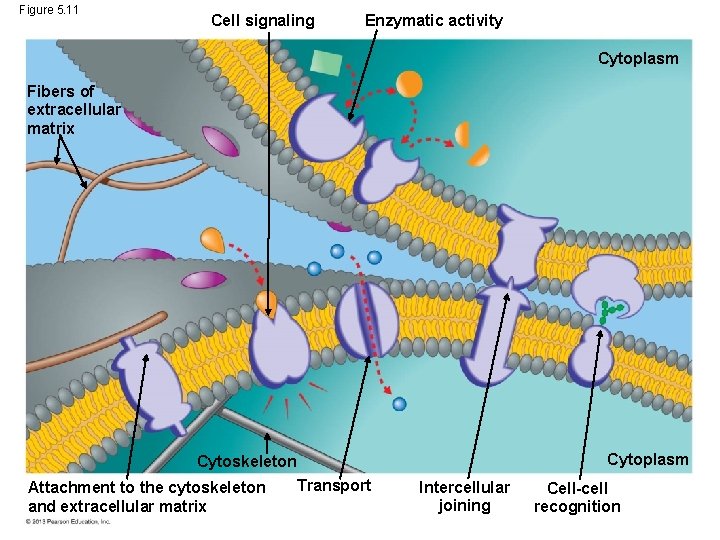
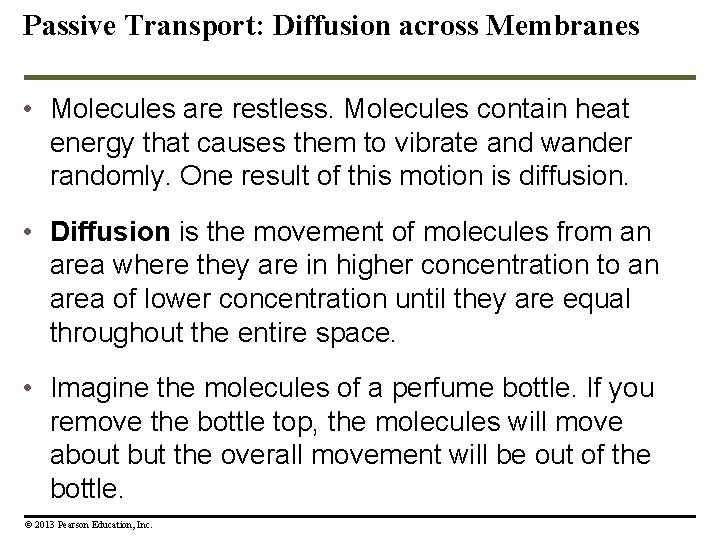
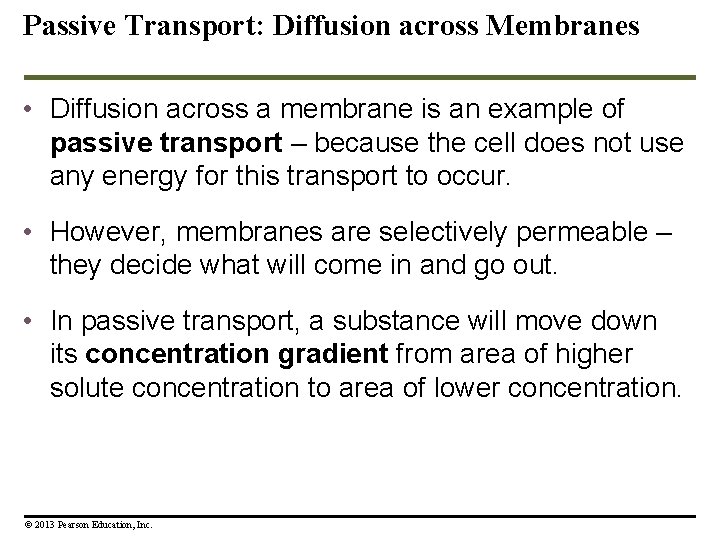
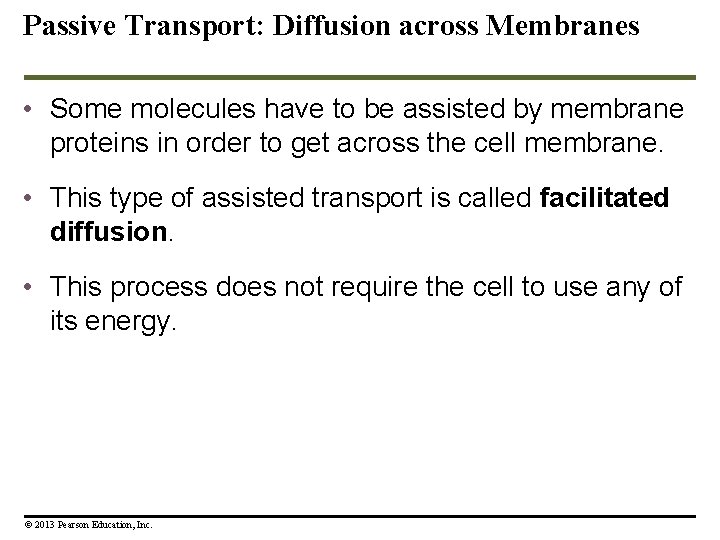
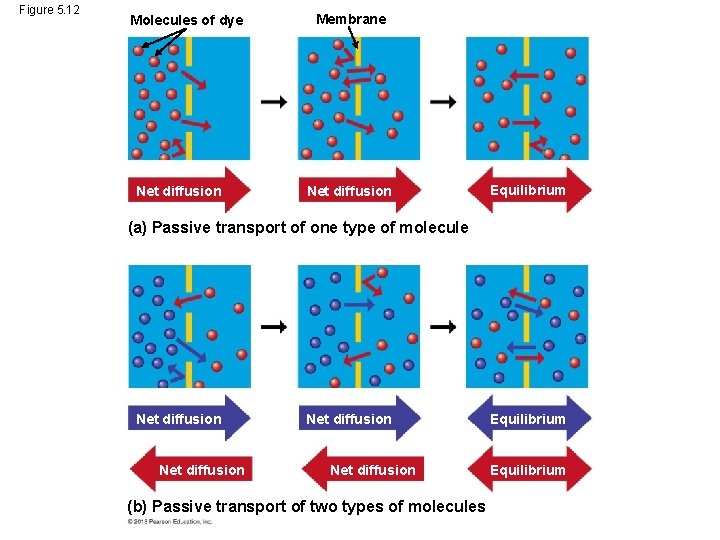
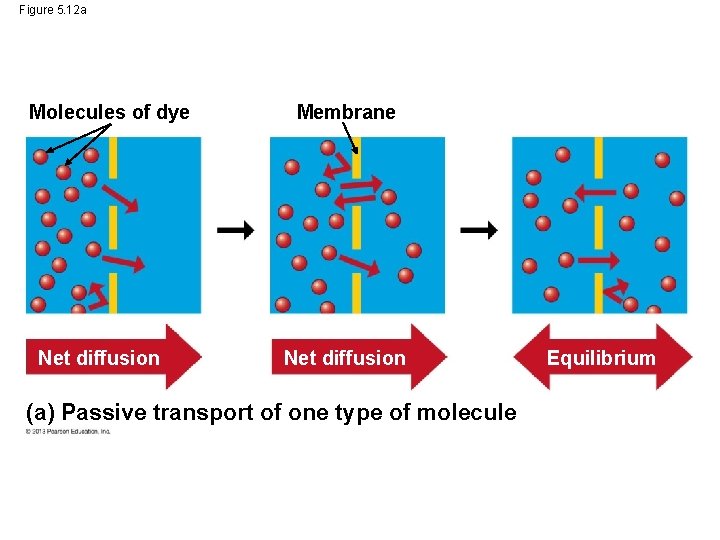
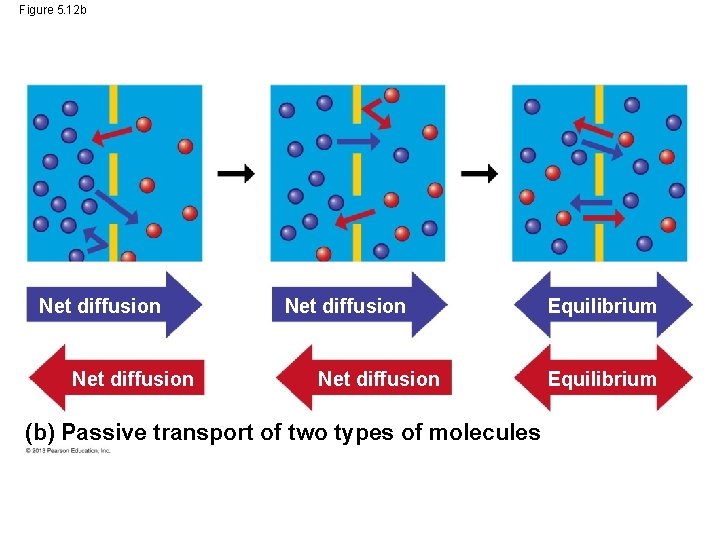
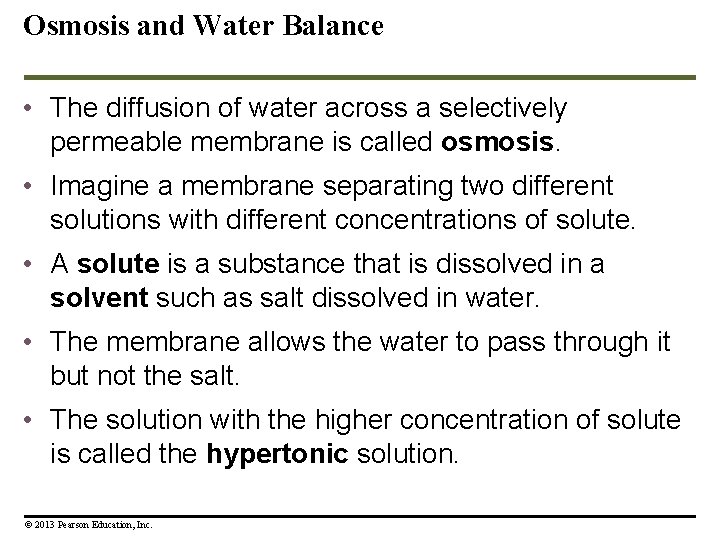
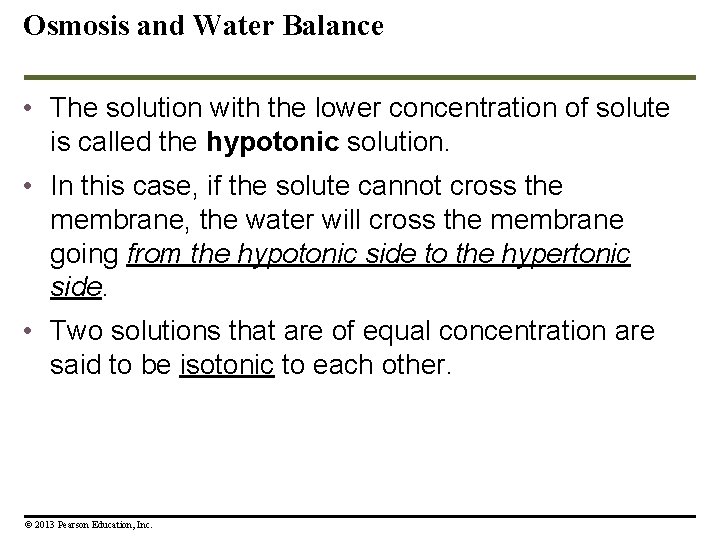
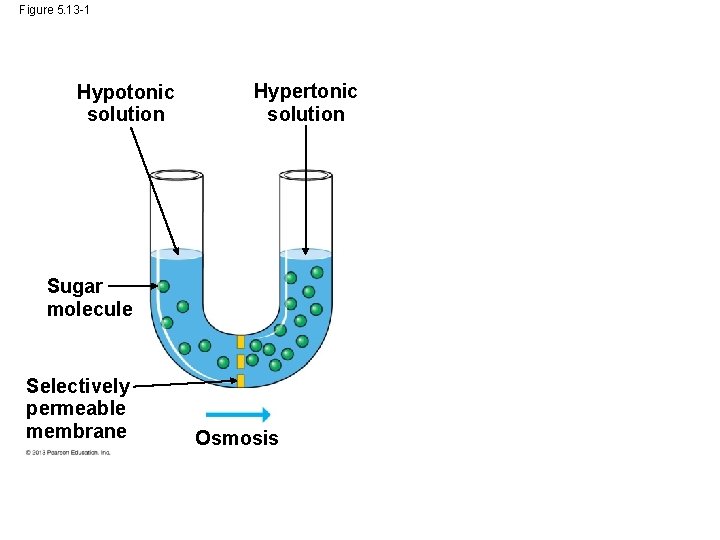
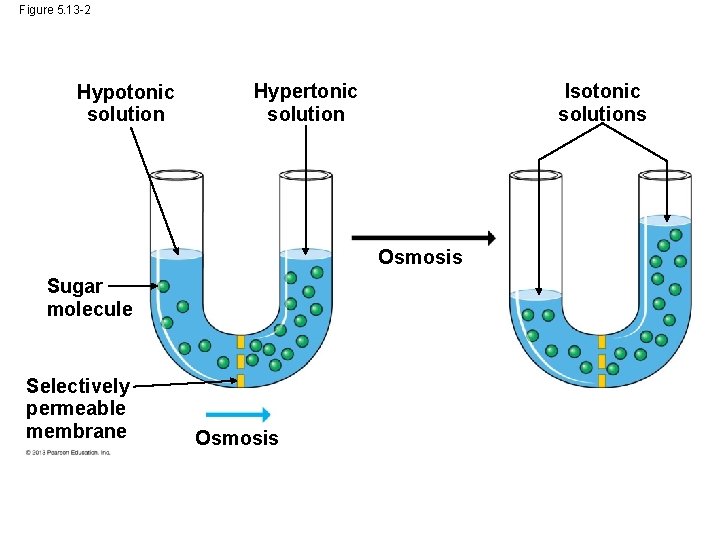
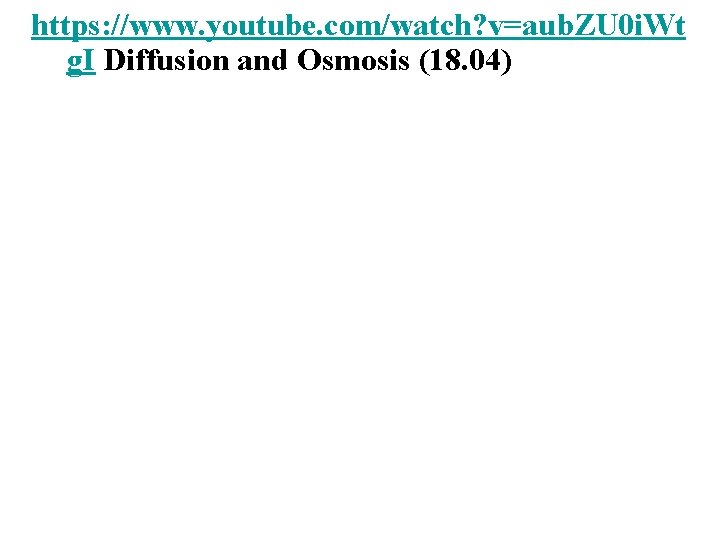
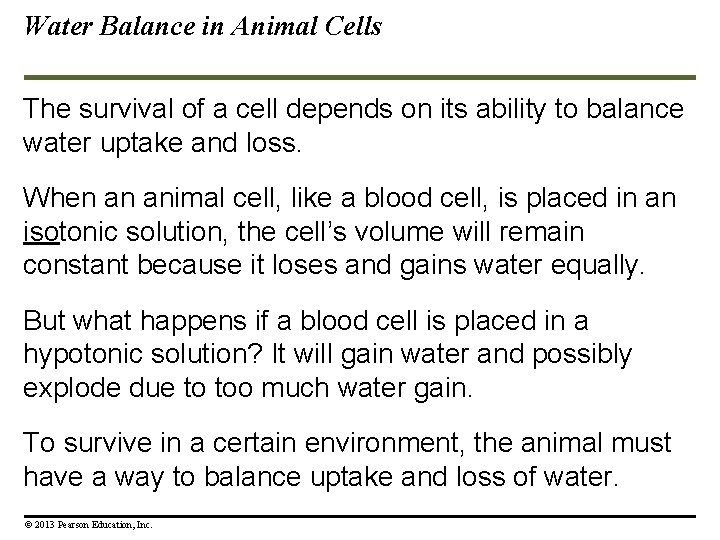
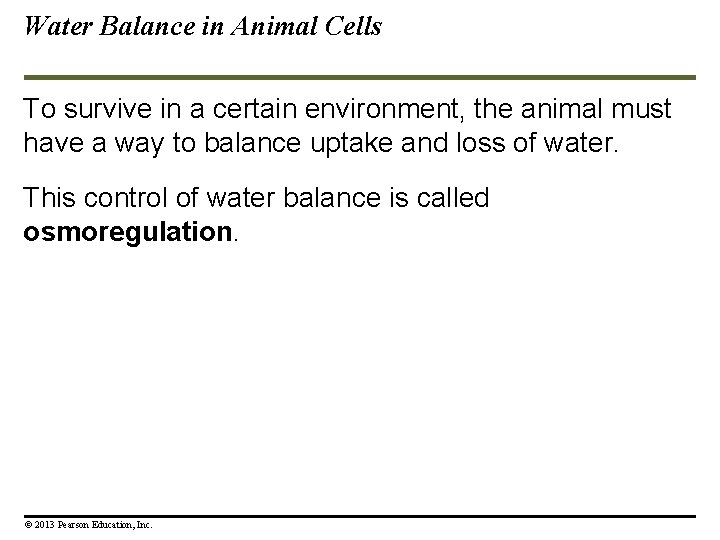
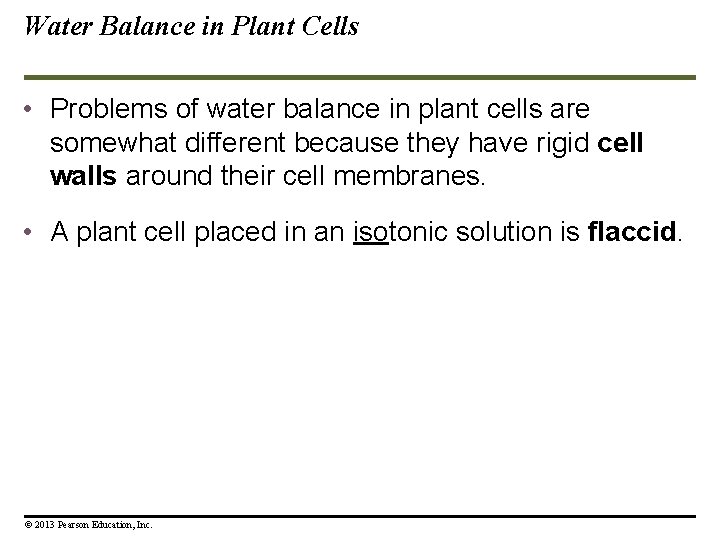
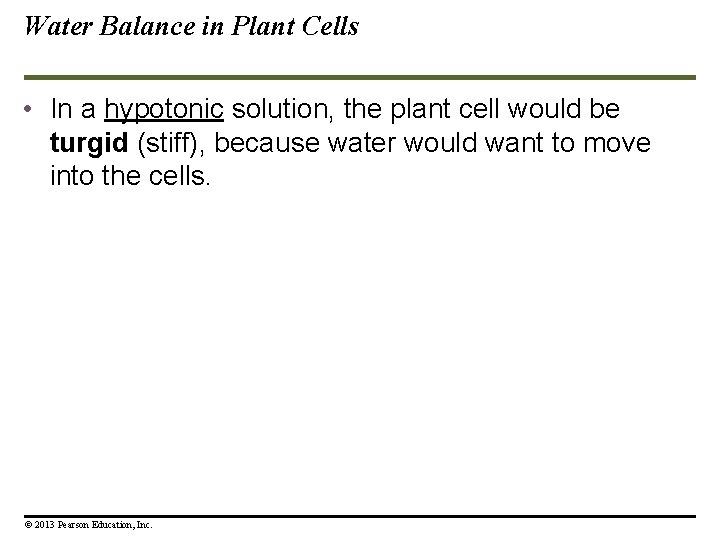
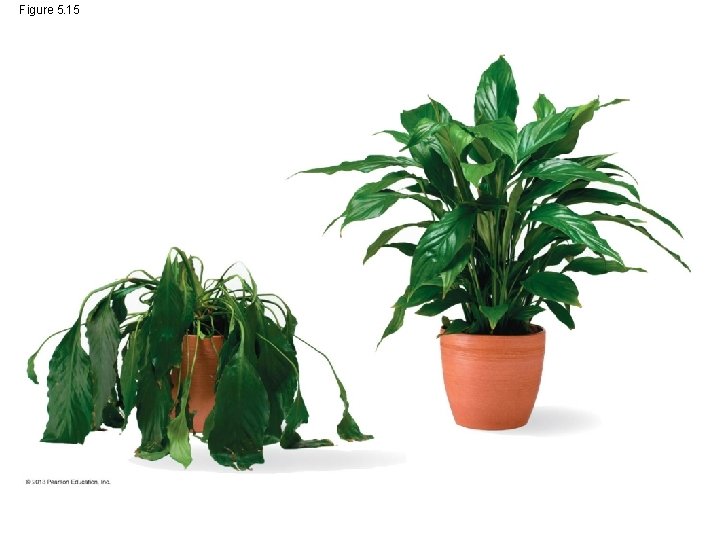
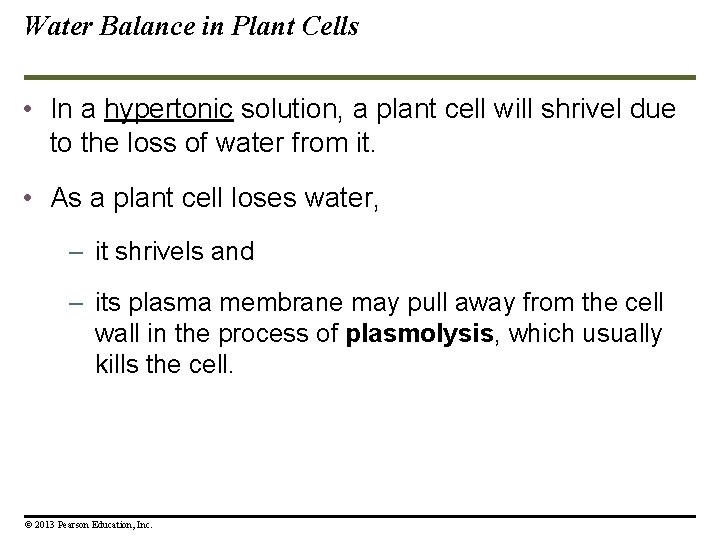
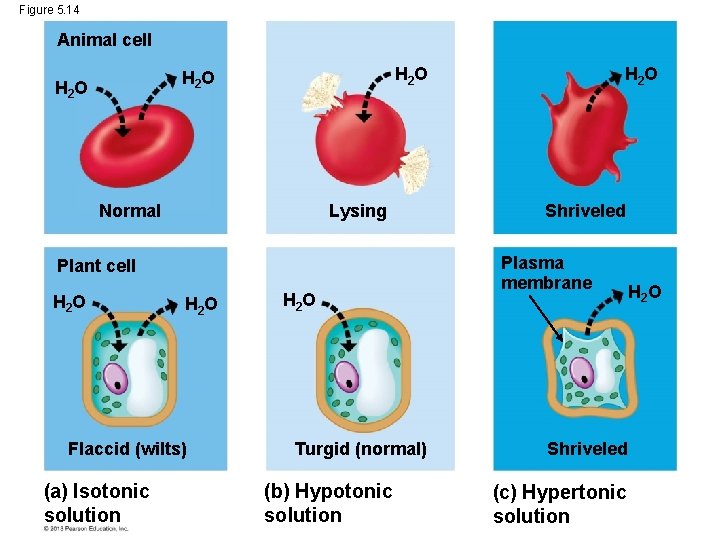
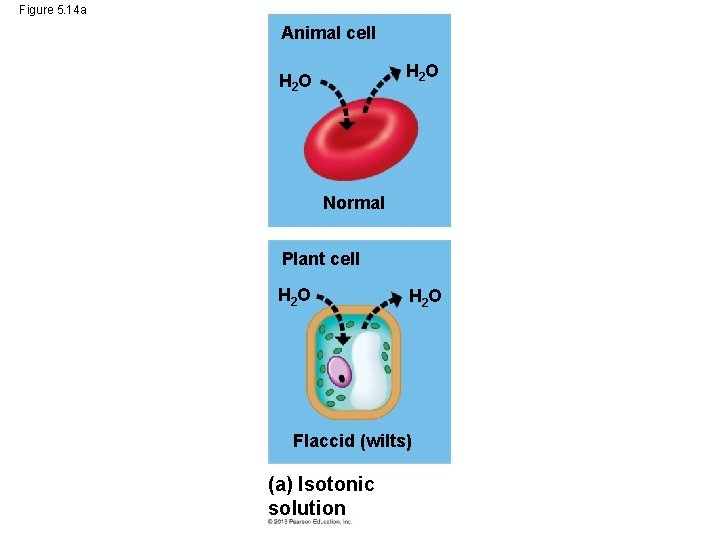
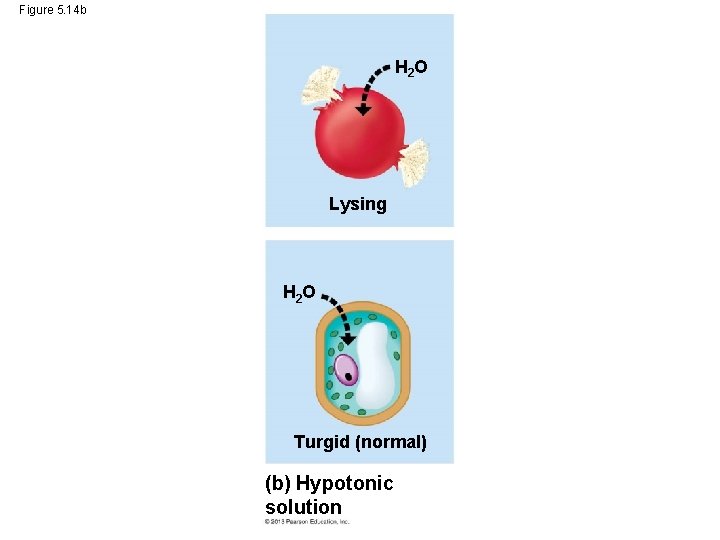
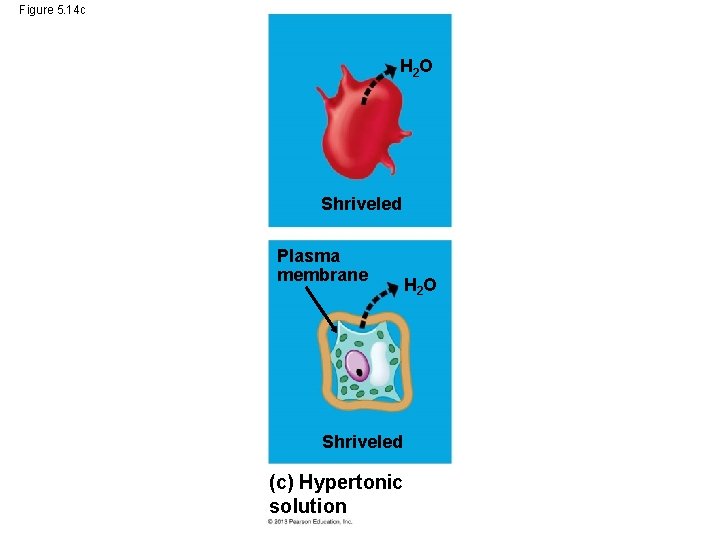
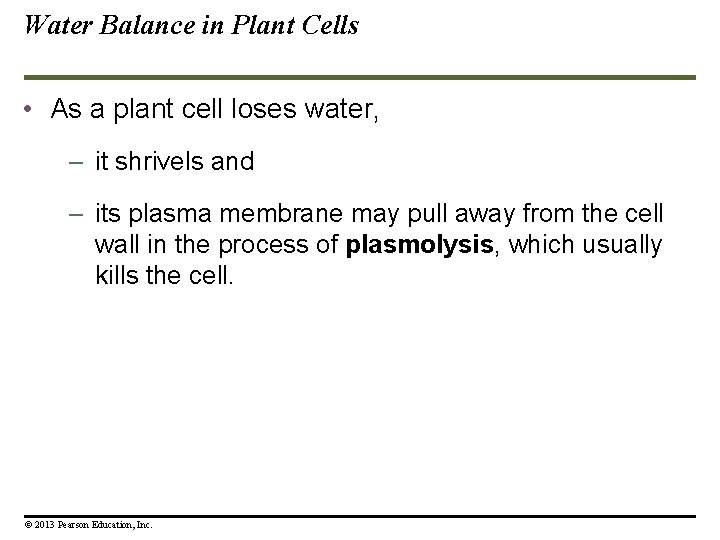
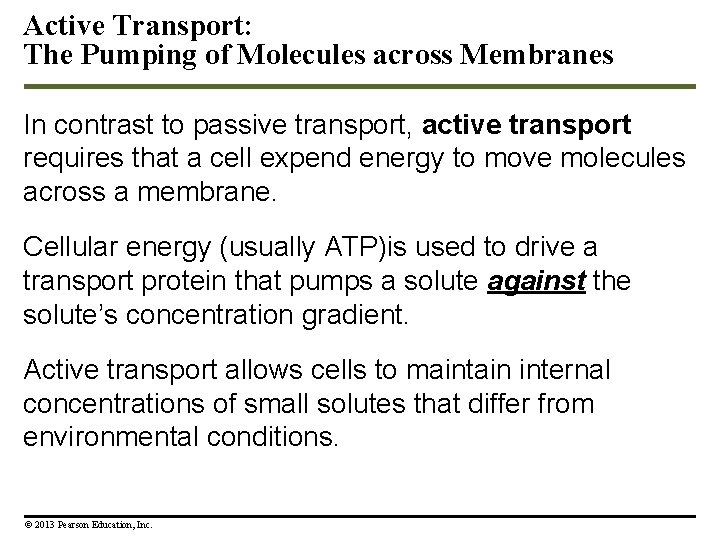
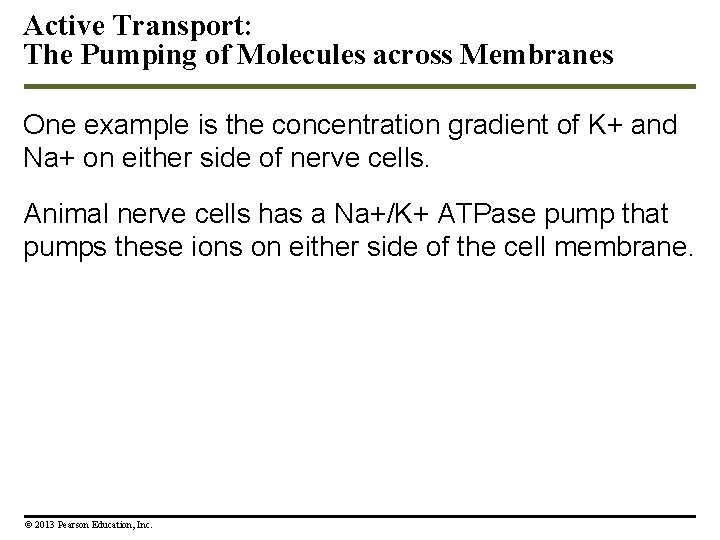
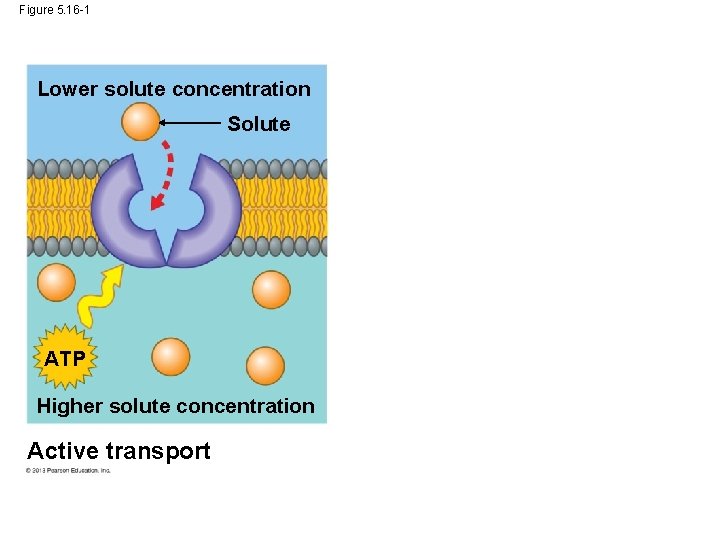
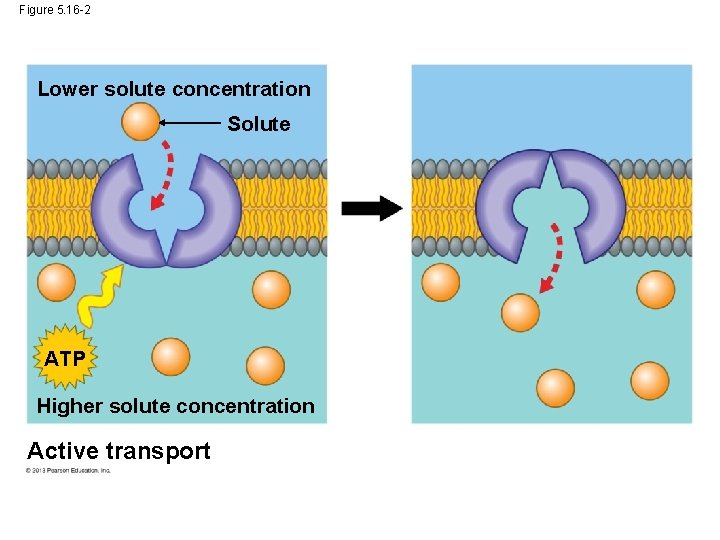
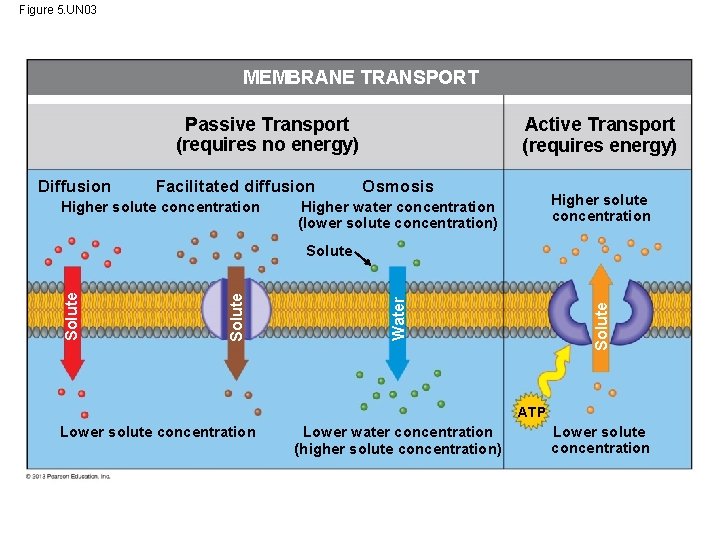
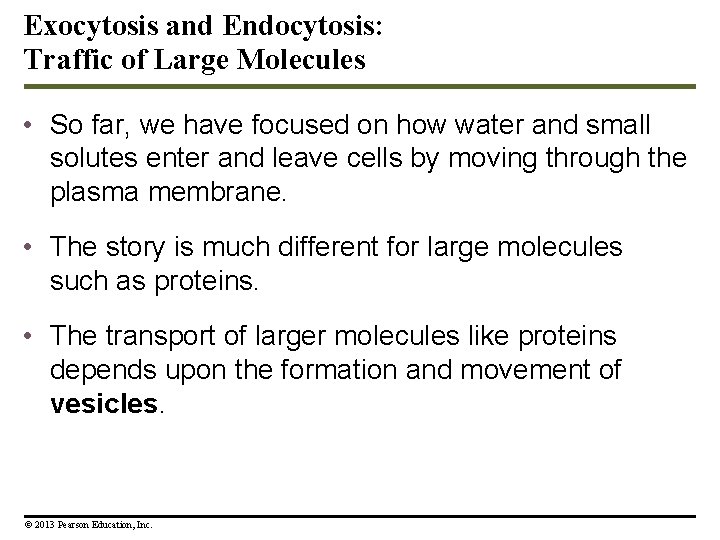
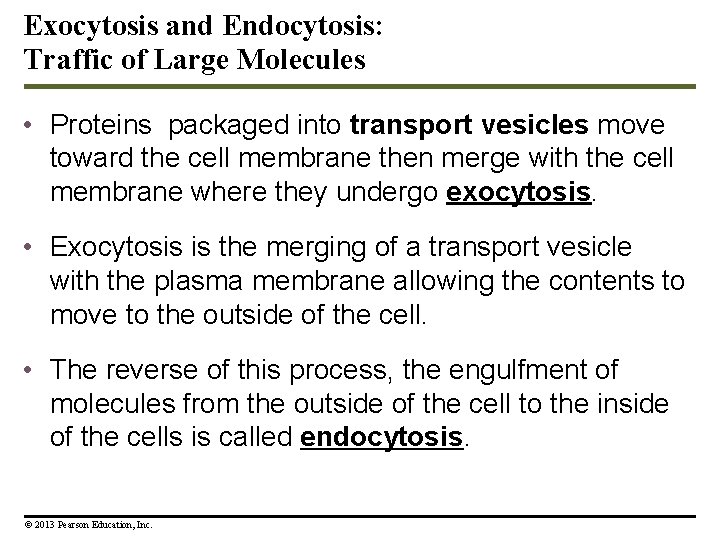
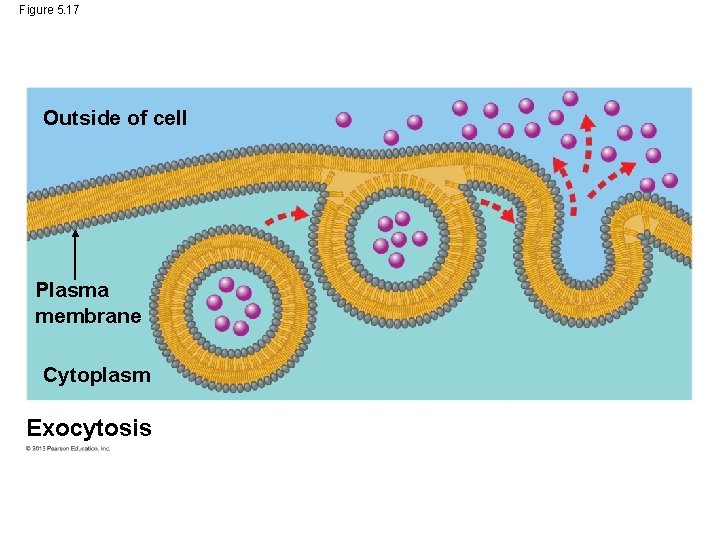
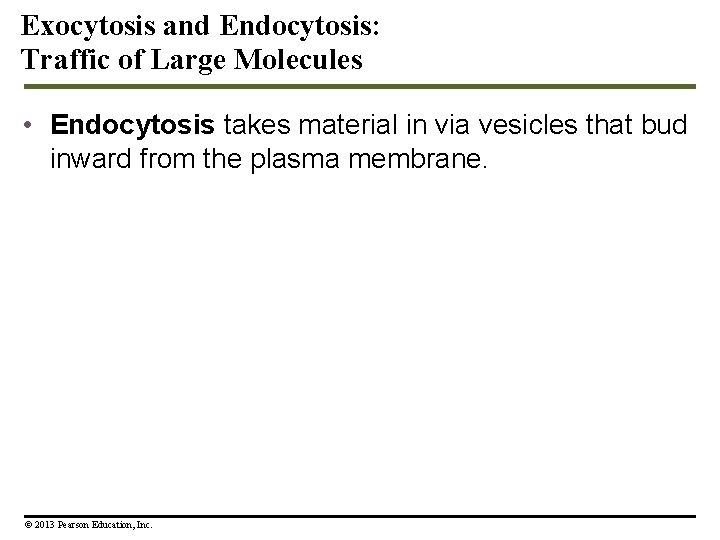
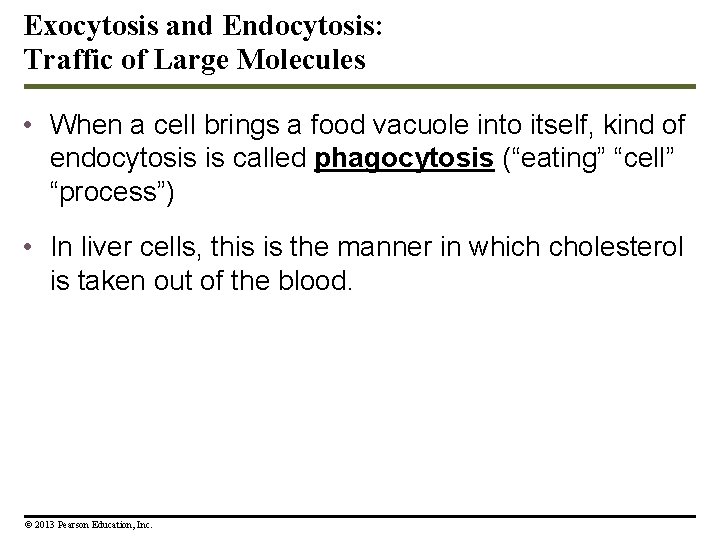
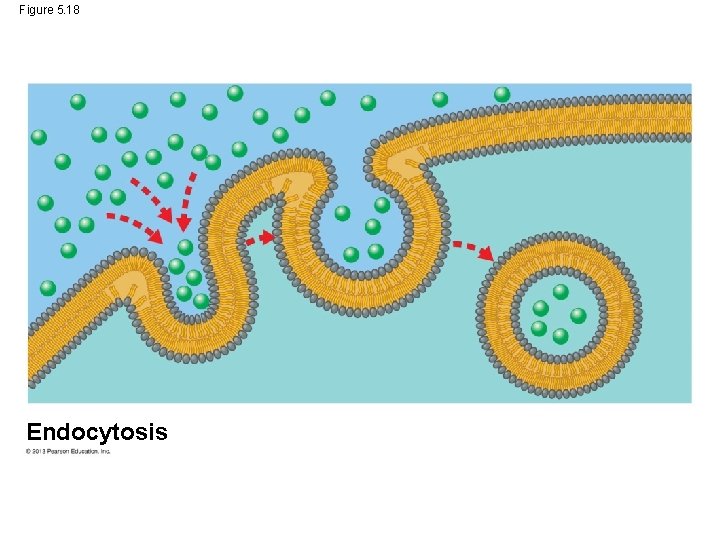
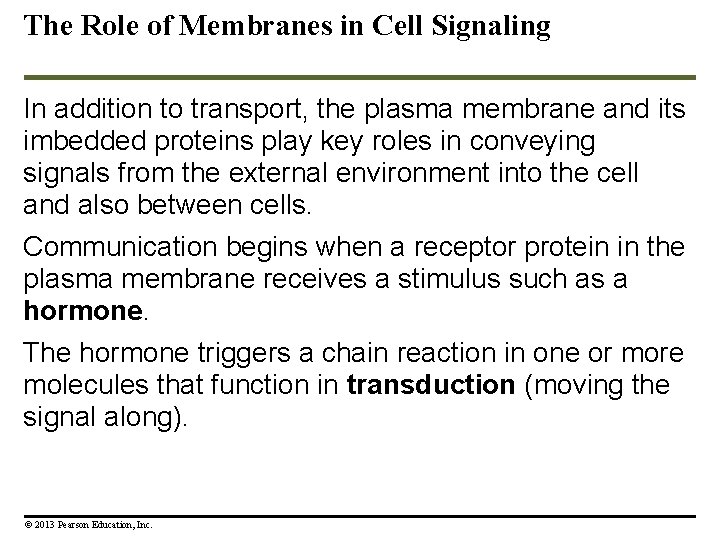
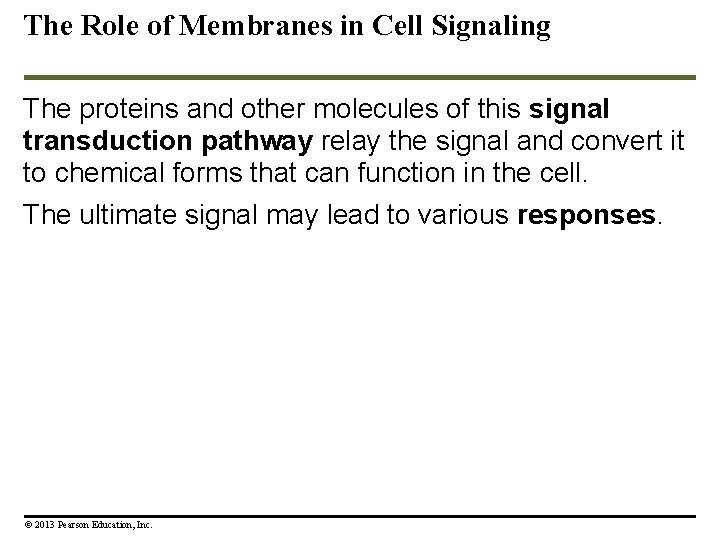
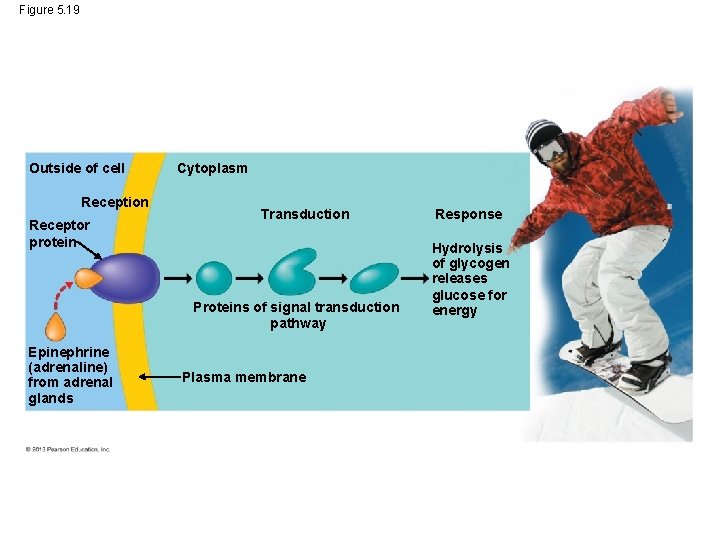
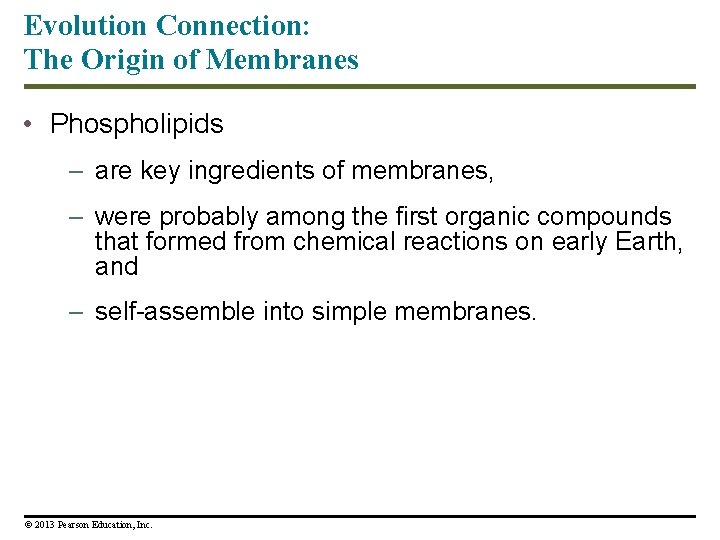
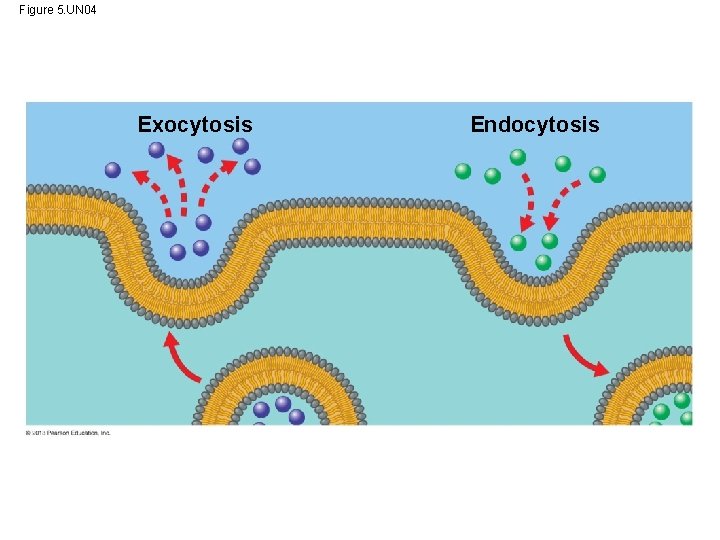
- Slides: 100
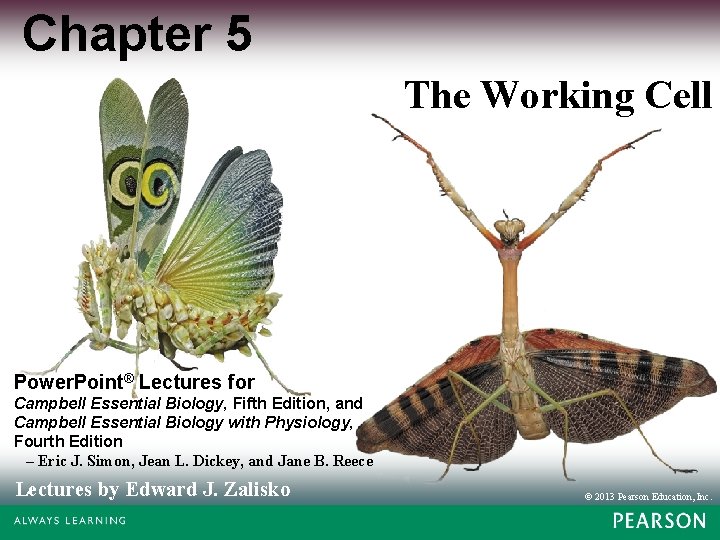
Chapter 5 The Working Cell Power. Point® Lectures for Campbell Essential Biology, Fifth Edition, and Campbell Essential Biology with Physiology, Fourth Edition – Eric J. Simon, Jean L. Dickey, and Jane B. Reece Lectures by Edward J. Zalisko © 2013 Pearson Education, Inc.
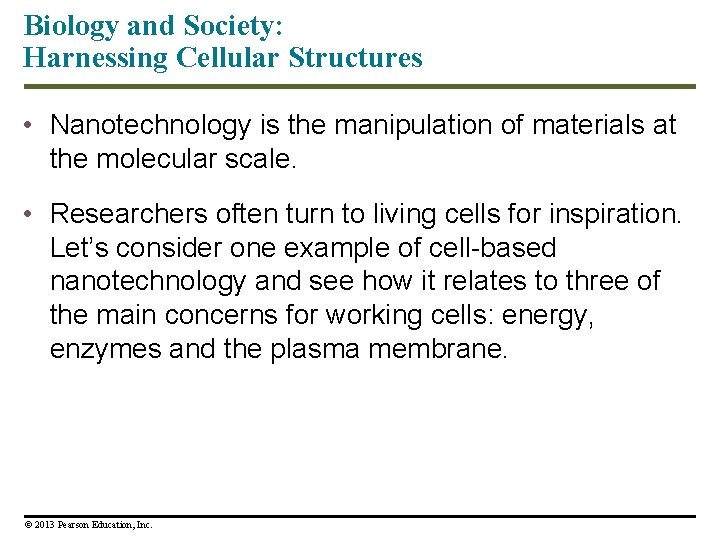
Biology and Society: Harnessing Cellular Structures • Nanotechnology is the manipulation of materials at the molecular scale. • Researchers often turn to living cells for inspiration. Let’s consider one example of cell-based nanotechnology and see how it relates to three of the main concerns for working cells: energy, enzymes and the plasma membrane. © 2013 Pearson Education, Inc.
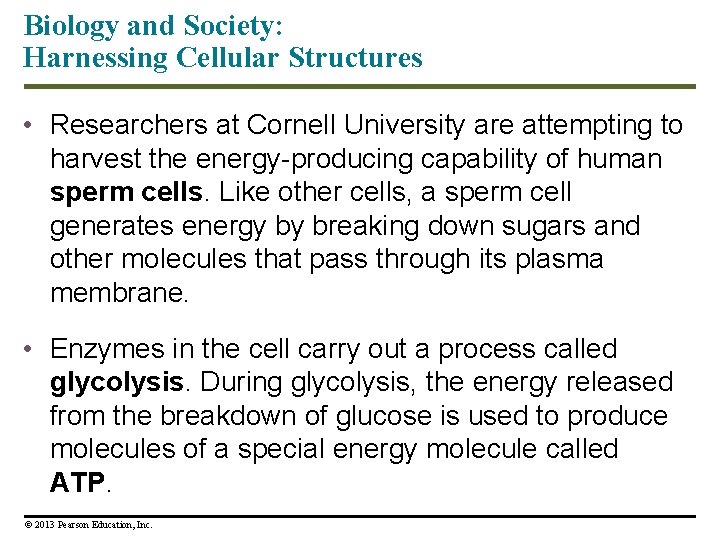
Biology and Society: Harnessing Cellular Structures • Researchers at Cornell University are attempting to harvest the energy-producing capability of human sperm cells. Like other cells, a sperm cell generates energy by breaking down sugars and other molecules that pass through its plasma membrane. • Enzymes in the cell carry out a process called glycolysis. During glycolysis, the energy released from the breakdown of glucose is used to produce molecules of a special energy molecule called ATP. © 2013 Pearson Education, Inc.
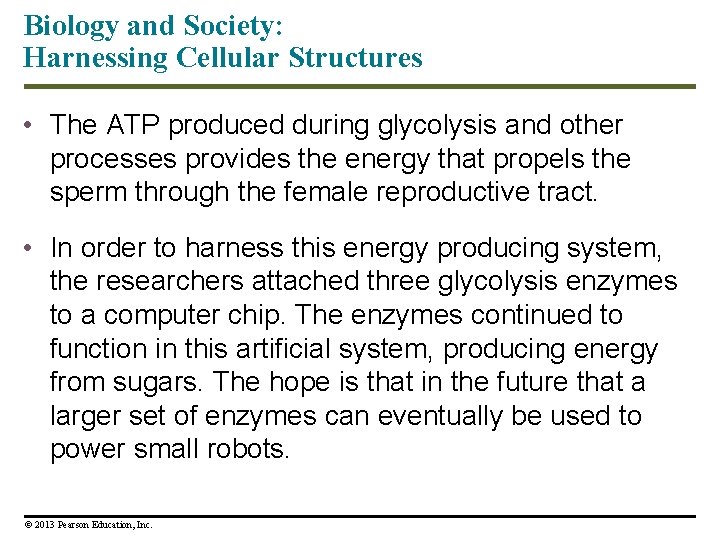
Biology and Society: Harnessing Cellular Structures • The ATP produced during glycolysis and other processes provides the energy that propels the sperm through the female reproductive tract. • In order to harness this energy producing system, the researchers attached three glycolysis enzymes to a computer chip. The enzymes continued to function in this artificial system, producing energy from sugars. The hope is that in the future that a larger set of enzymes can eventually be used to power small robots. © 2013 Pearson Education, Inc.
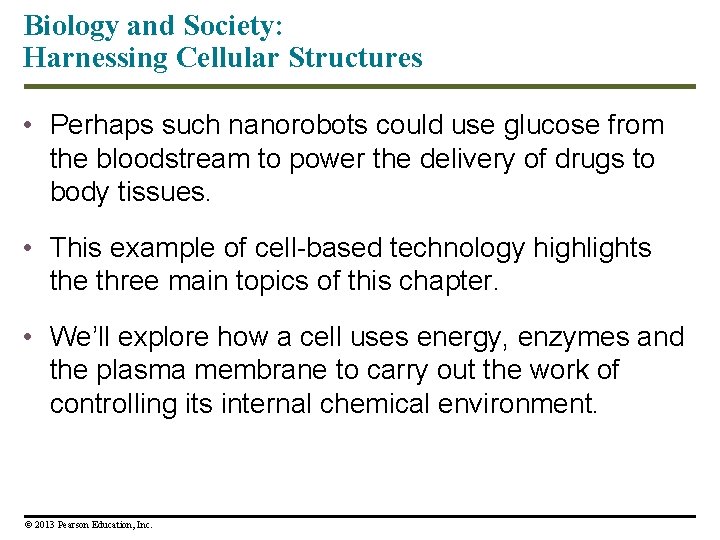
Biology and Society: Harnessing Cellular Structures • Perhaps such nanorobots could use glucose from the bloodstream to power the delivery of drugs to body tissues. • This example of cell-based technology highlights the three main topics of this chapter. • We’ll explore how a cell uses energy, enzymes and the plasma membrane to carry out the work of controlling its internal chemical environment. © 2013 Pearson Education, Inc.
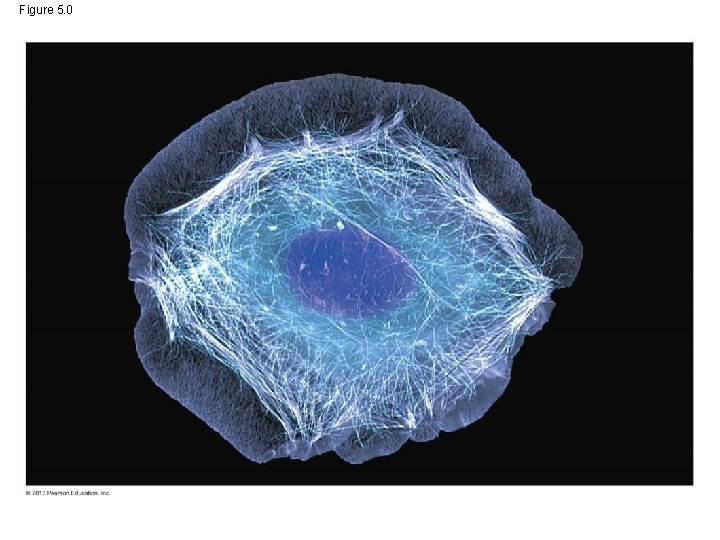
Figure 5. 0
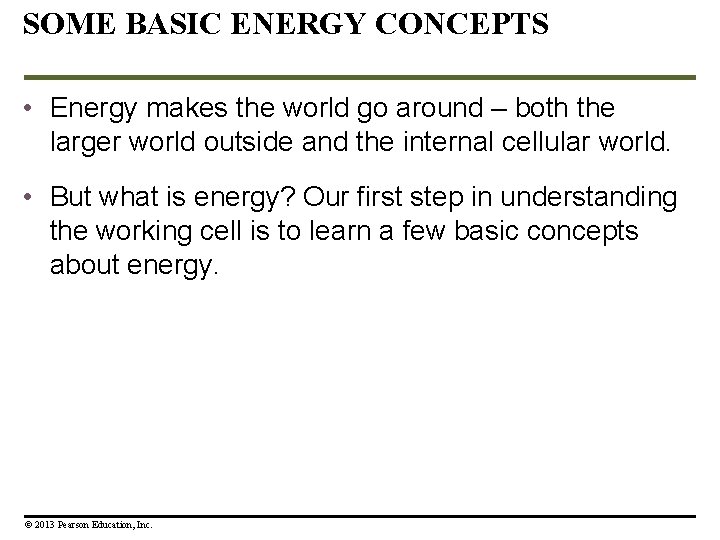
SOME BASIC ENERGY CONCEPTS • Energy makes the world go around – both the larger world outside and the internal cellular world. • But what is energy? Our first step in understanding the working cell is to learn a few basic concepts about energy. © 2013 Pearson Education, Inc.
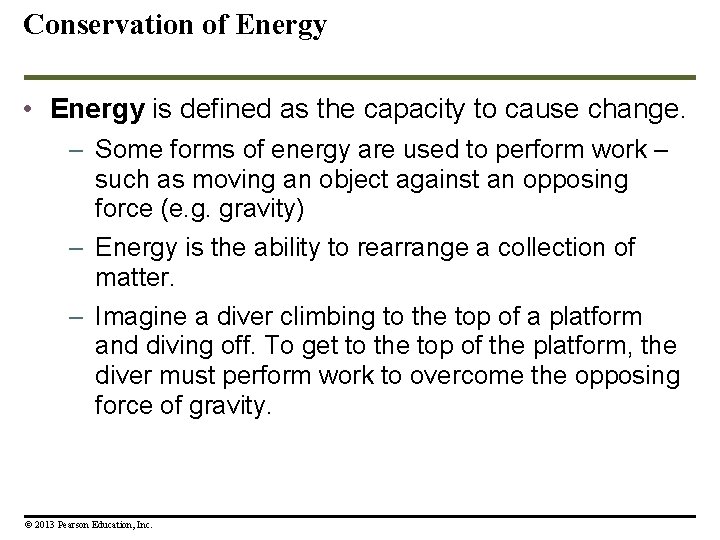
Conservation of Energy • Energy is defined as the capacity to cause change. – Some forms of energy are used to perform work – such as moving an object against an opposing force (e. g. gravity) – Energy is the ability to rearrange a collection of matter. – Imagine a diver climbing to the top of a platform and diving off. To get to the top of the platform, the diver must perform work to overcome the opposing force of gravity. © 2013 Pearson Education, Inc.
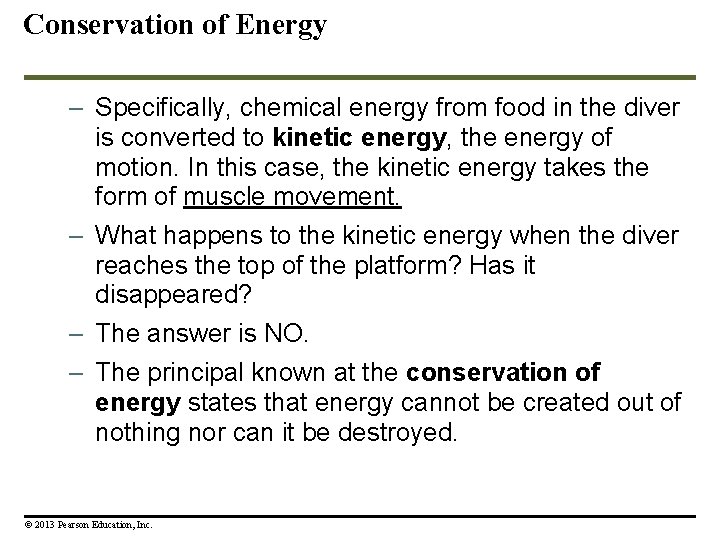
Conservation of Energy – Specifically, chemical energy from food in the diver is converted to kinetic energy, the energy of motion. In this case, the kinetic energy takes the form of muscle movement. – What happens to the kinetic energy when the diver reaches the top of the platform? Has it disappeared? – The answer is NO. – The principal known at the conservation of energy states that energy cannot be created out of nothing nor can it be destroyed. © 2013 Pearson Education, Inc.
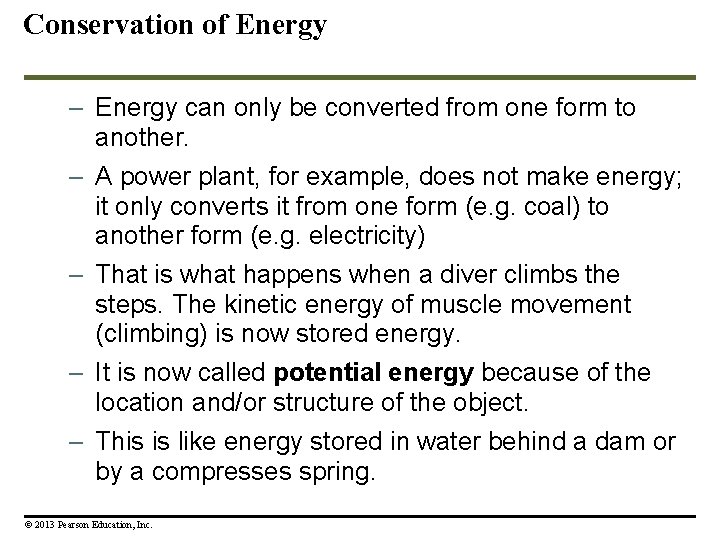
Conservation of Energy – Energy can only be converted from one form to another. – A power plant, for example, does not make energy; it only converts it from one form (e. g. coal) to another form (e. g. electricity) – That is what happens when a diver climbs the steps. The kinetic energy of muscle movement (climbing) is now stored energy. – It is now called potential energy because of the location and/or structure of the object. – This is like energy stored in water behind a dam or by a compresses spring. © 2013 Pearson Education, Inc.
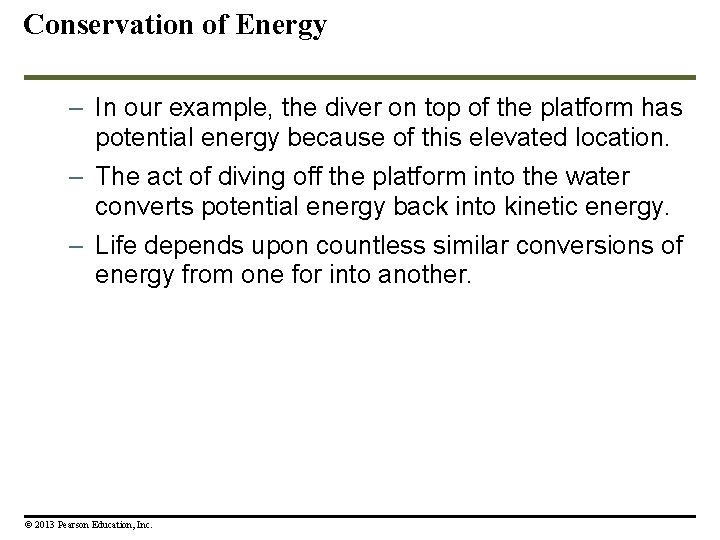
Conservation of Energy – In our example, the diver on top of the platform has potential energy because of this elevated location. – The act of diving off the platform into the water converts potential energy back into kinetic energy. – Life depends upon countless similar conversions of energy from one for into another. © 2013 Pearson Education, Inc.
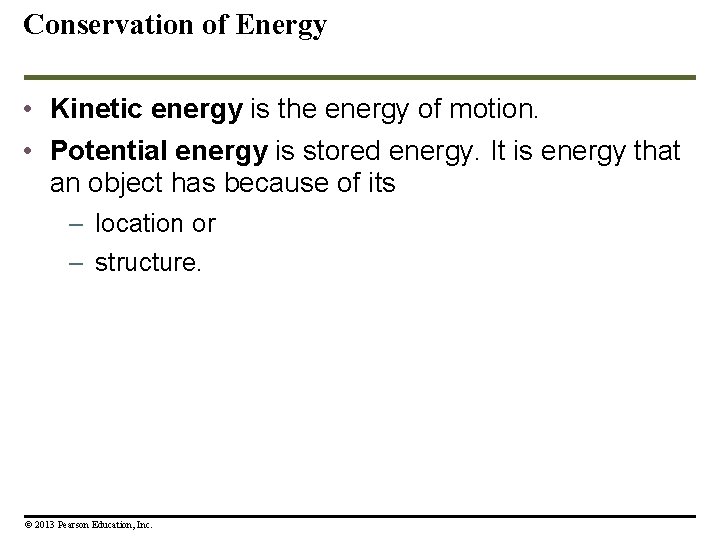
Conservation of Energy • Kinetic energy is the energy of motion. • Potential energy is stored energy. It is energy that an object has because of its – location or – structure. © 2013 Pearson Education, Inc.
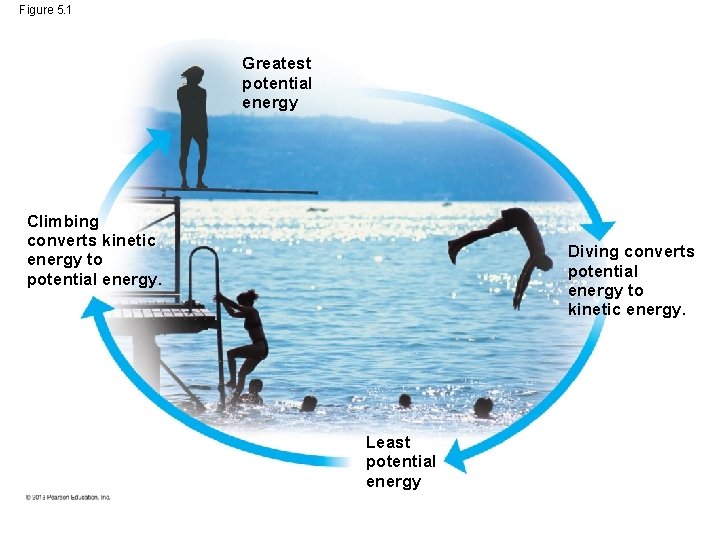
Figure 5. 1 Greatest potential energy Climbing converts kinetic energy to potential energy. Diving converts potential energy to kinetic energy. Least potential energy
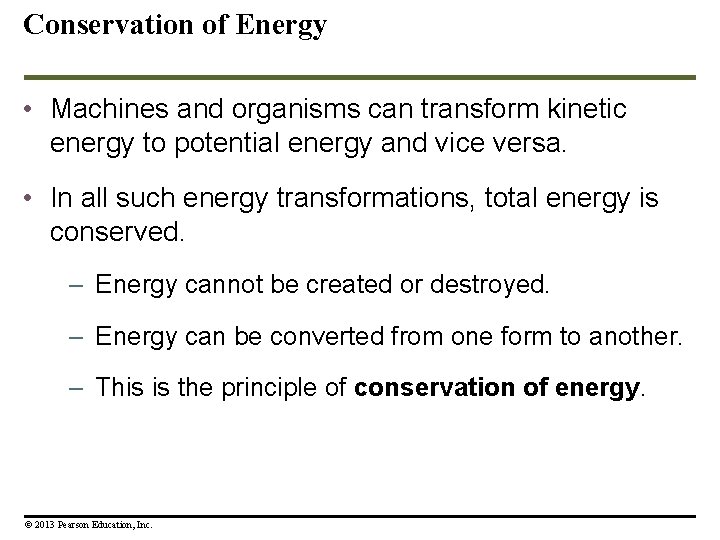
Conservation of Energy • Machines and organisms can transform kinetic energy to potential energy and vice versa. • In all such energy transformations, total energy is conserved. – Energy cannot be created or destroyed. – Energy can be converted from one form to another. – This is the principle of conservation of energy. © 2013 Pearson Education, Inc.
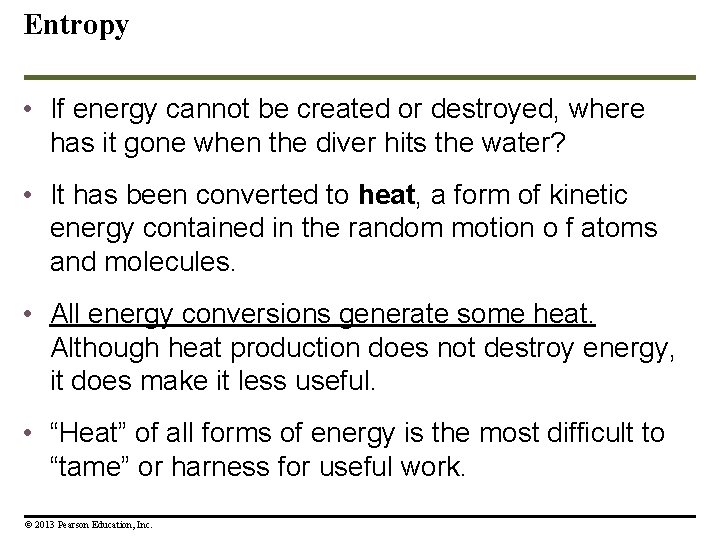
Entropy • If energy cannot be created or destroyed, where has it gone when the diver hits the water? • It has been converted to heat, a form of kinetic energy contained in the random motion o f atoms and molecules. • All energy conversions generate some heat. Although heat production does not destroy energy, it does make it less useful. • “Heat” of all forms of energy is the most difficult to “tame” or harness for useful work. © 2013 Pearson Education, Inc.
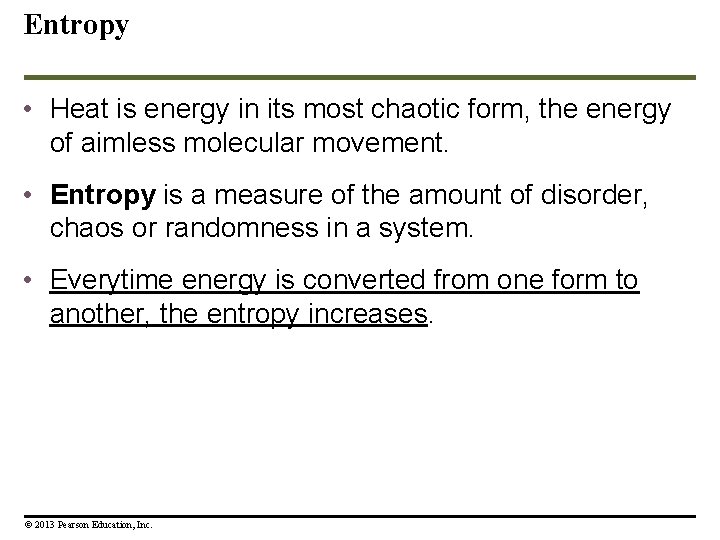
Entropy • Heat is energy in its most chaotic form, the energy of aimless molecular movement. • Entropy is a measure of the amount of disorder, chaos or randomness in a system. • Everytime energy is converted from one form to another, the entropy increases. © 2013 Pearson Education, Inc.
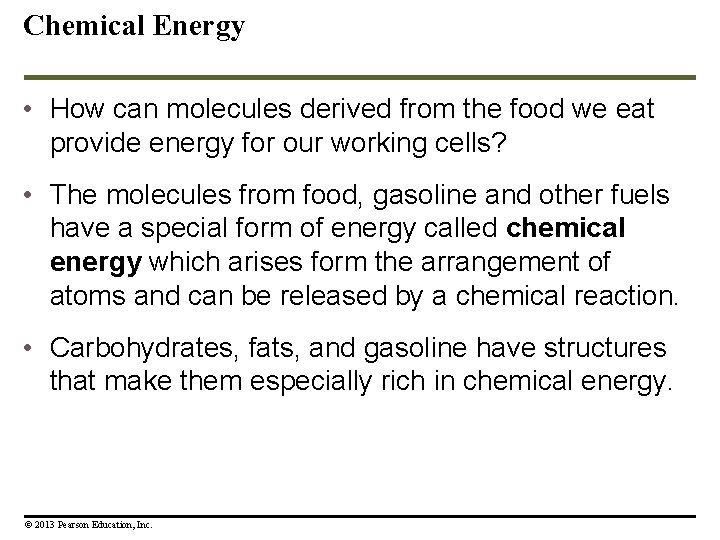
Chemical Energy • How can molecules derived from the food we eat provide energy for our working cells? • The molecules from food, gasoline and other fuels have a special form of energy called chemical energy which arises form the arrangement of atoms and can be released by a chemical reaction. • Carbohydrates, fats, and gasoline have structures that make them especially rich in chemical energy. © 2013 Pearson Education, Inc.
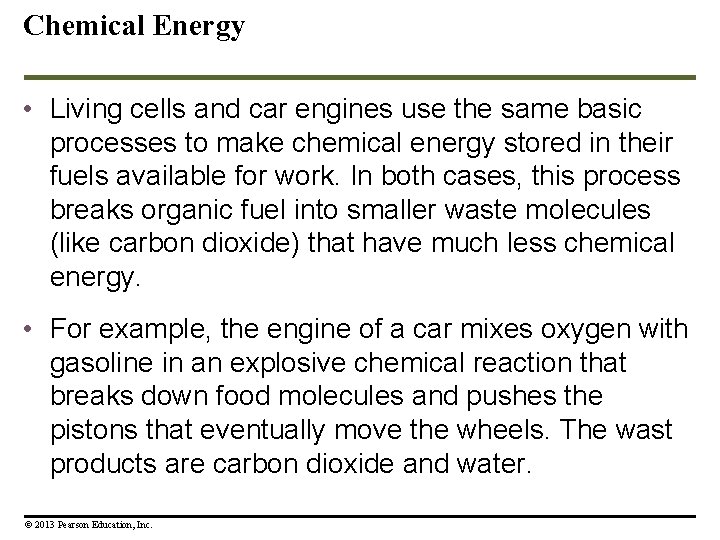
Chemical Energy • Living cells and car engines use the same basic processes to make chemical energy stored in their fuels available for work. In both cases, this process breaks organic fuel into smaller waste molecules (like carbon dioxide) that have much less chemical energy. • For example, the engine of a car mixes oxygen with gasoline in an explosive chemical reaction that breaks down food molecules and pushes the pistons that eventually move the wheels. The wast products are carbon dioxide and water. © 2013 Pearson Education, Inc.
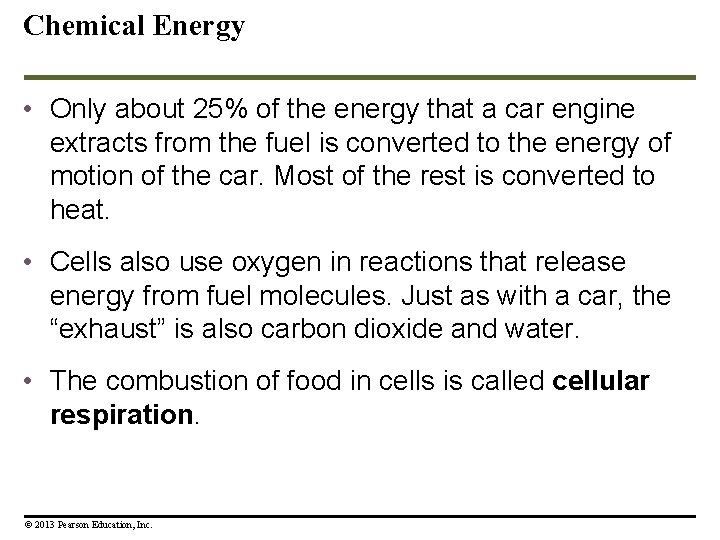
Chemical Energy • Only about 25% of the energy that a car engine extracts from the fuel is converted to the energy of motion of the car. Most of the rest is converted to heat. • Cells also use oxygen in reactions that release energy from fuel molecules. Just as with a car, the “exhaust” is also carbon dioxide and water. • The combustion of food in cells is called cellular respiration. © 2013 Pearson Education, Inc.
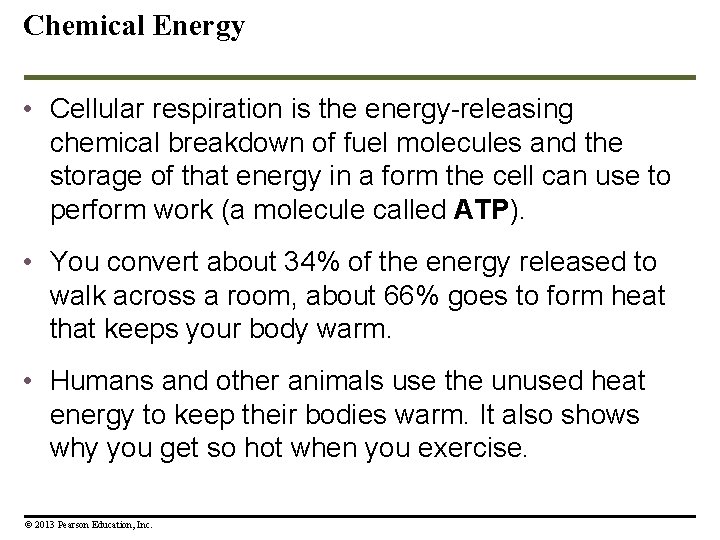
Chemical Energy • Cellular respiration is the energy-releasing chemical breakdown of fuel molecules and the storage of that energy in a form the cell can use to perform work (a molecule called ATP). • You convert about 34% of the energy released to walk across a room, about 66% goes to form heat that keeps your body warm. • Humans and other animals use the unused heat energy to keep their bodies warm. It also shows why you get so hot when you exercise. © 2013 Pearson Education, Inc.
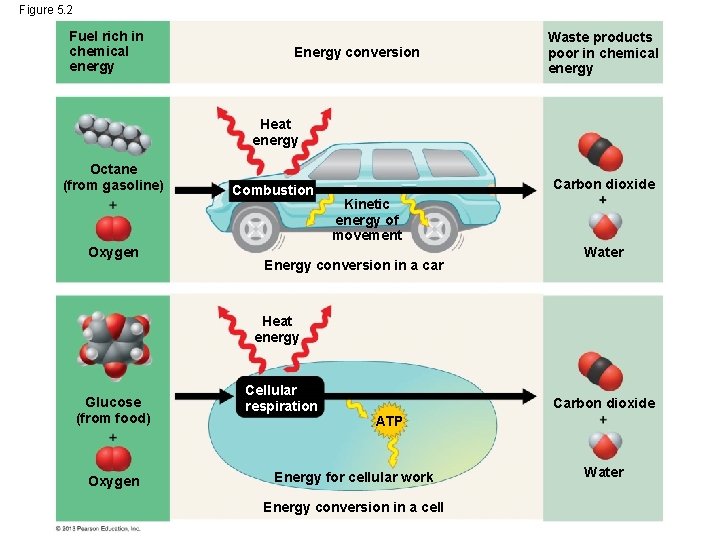
Figure 5. 2 Fuel rich in chemical energy Energy conversion Waste products poor in chemical energy Heat energy Octane (from gasoline) Oxygen Combustion Carbon dioxide Kinetic energy of movement Energy conversion in a car Water Heat energy Glucose (from food) Oxygen Cellular respiration Carbon dioxide ATP Energy for cellular work Energy conversion in a cell Water
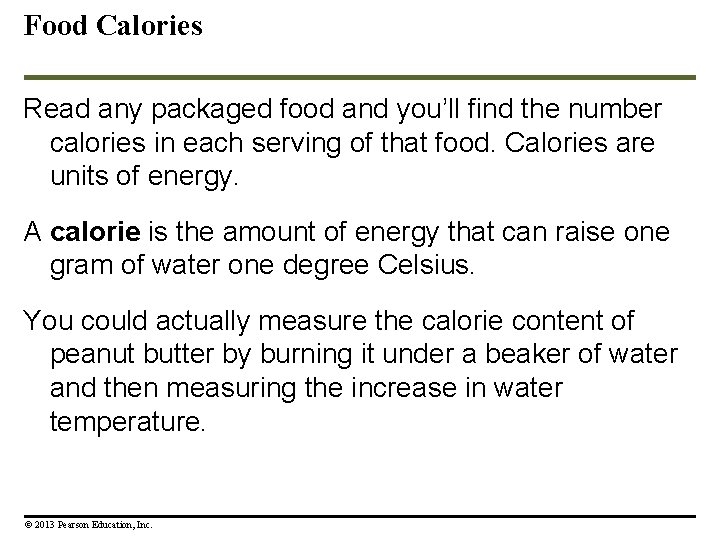
Food Calories Read any packaged food and you’ll find the number calories in each serving of that food. Calories are units of energy. A calorie is the amount of energy that can raise one gram of water one degree Celsius. You could actually measure the calorie content of peanut butter by burning it under a beaker of water and then measuring the increase in water temperature. © 2013 Pearson Education, Inc.
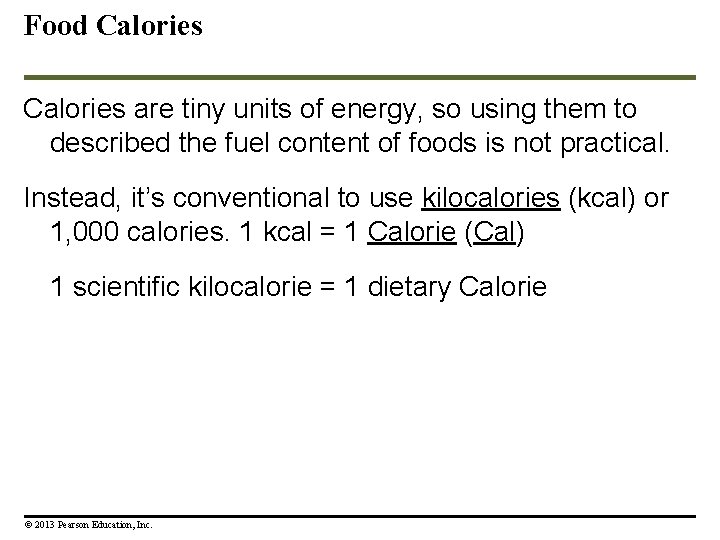
Food Calories are tiny units of energy, so using them to described the fuel content of foods is not practical. Instead, it’s conventional to use kilocalories (kcal) or 1, 000 calories. 1 kcal = 1 Calorie (Cal) 1 scientific kilocalorie = 1 dietary Calorie © 2013 Pearson Education, Inc.
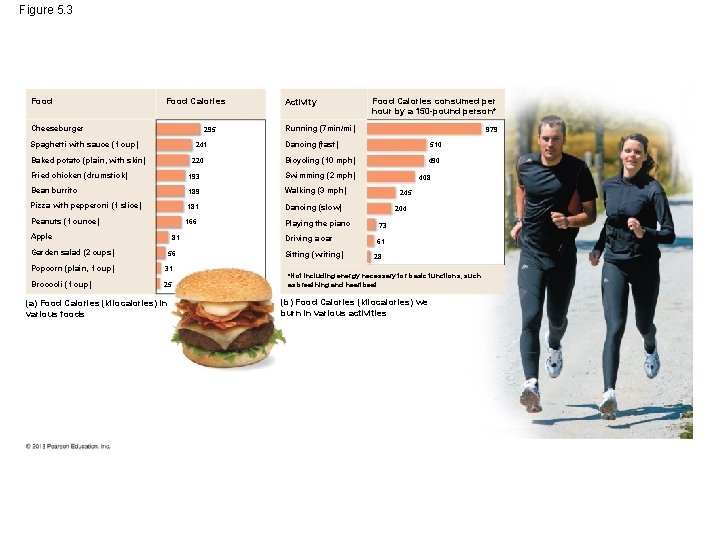
Figure 5. 3 Food Calories Cheeseburger 295 Spaghetti with sauce (1 cup) 241 Baked potato (plain, with skin) 220 Activity Running (7 min/mi) 510 Bicycling (10 mph) 490 193 Swimming (2 mph) Bean burrito 189 Walking (3 mph) 181 Dancing (slow) Peanuts (1 ounce) 166 Apple 81 Garden salad (2 cups) 56 Popcorn (plain, 1 cup) 31 Broccoli (1 cup) 25 (a) Food Calories (kilocalories) in various foods 979 Dancing (fast) Fried chicken (drumstick) Pizza with pepperoni (1 slice) Food Calories consumed per hour by a 150 -pound person* 408 245 204 Playing the piano 73 Driving a car 61 Sitting (writing) 28 *Not including energy necessary for basic functions, such as breathing and heartbeat (b) Food Calories (kilocalories) we burn in various activities
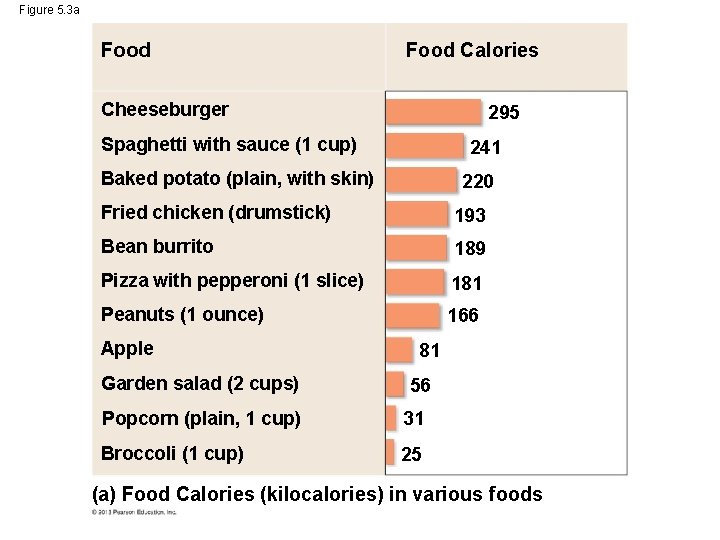
Figure 5. 3 a Food Calories Cheeseburger 295 Spaghetti with sauce (1 cup) 241 Baked potato (plain, with skin) 220 Fried chicken (drumstick) 193 Bean burrito 189 Pizza with pepperoni (1 slice) 181 Peanuts (1 ounce) 166 Apple Garden salad (2 cups) 81 56 Popcorn (plain, 1 cup) 31 Broccoli (1 cup) 25 (a) Food Calories (kilocalories) in various foods
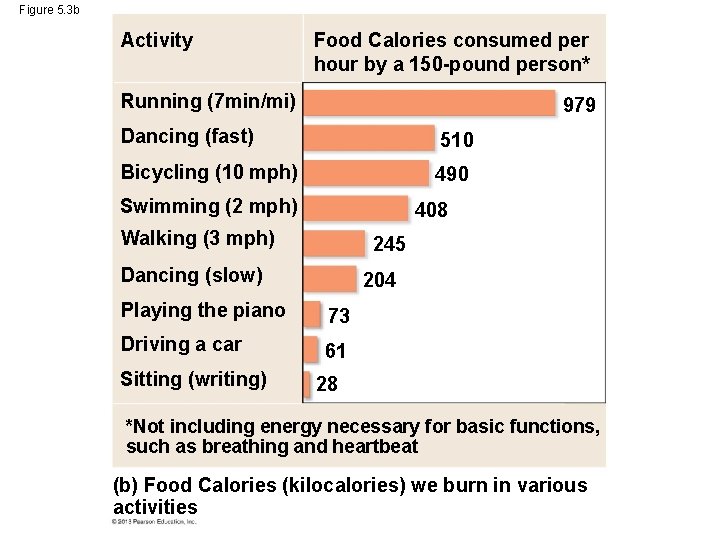
Figure 5. 3 b Activity Food Calories consumed per hour by a 150 -pound person* Running (7 min/mi) 979 Dancing (fast) 510 Bicycling (10 mph) 490 Swimming (2 mph) 408 Walking (3 mph) 245 Dancing (slow) 204 Playing the piano 73 Driving a car 61 Sitting (writing) 28 *Not including energy necessary for basic functions, such as breathing and heartbeat (b) Food Calories (kilocalories) we burn in various activities
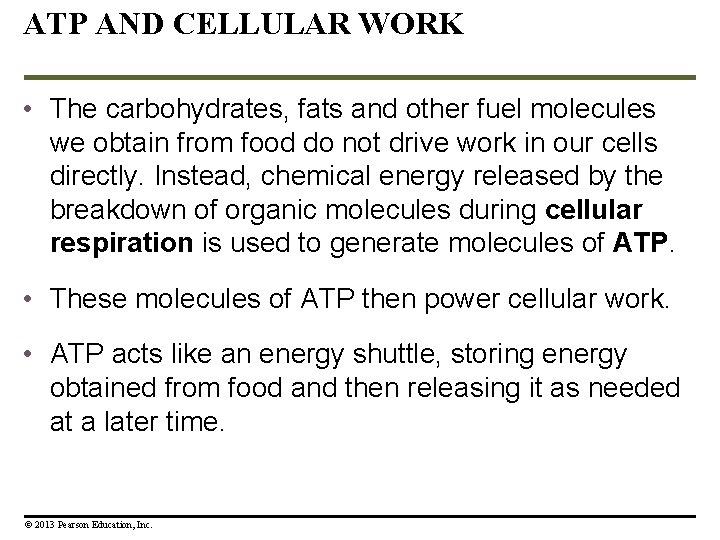
ATP AND CELLULAR WORK • The carbohydrates, fats and other fuel molecules we obtain from food do not drive work in our cells directly. Instead, chemical energy released by the breakdown of organic molecules during cellular respiration is used to generate molecules of ATP. • These molecules of ATP then power cellular work. • ATP acts like an energy shuttle, storing energy obtained from food and then releasing it as needed at a later time. © 2013 Pearson Education, Inc.
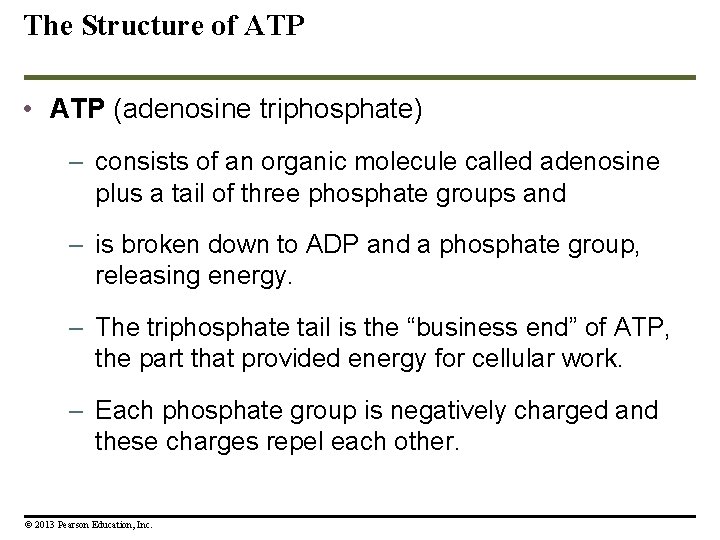
The Structure of ATP • ATP (adenosine triphosphate) – consists of an organic molecule called adenosine plus a tail of three phosphate groups and – is broken down to ADP and a phosphate group, releasing energy. – The triphosphate tail is the “business end” of ATP, the part that provided energy for cellular work. – Each phosphate group is negatively charged and these charges repel each other. © 2013 Pearson Education, Inc.
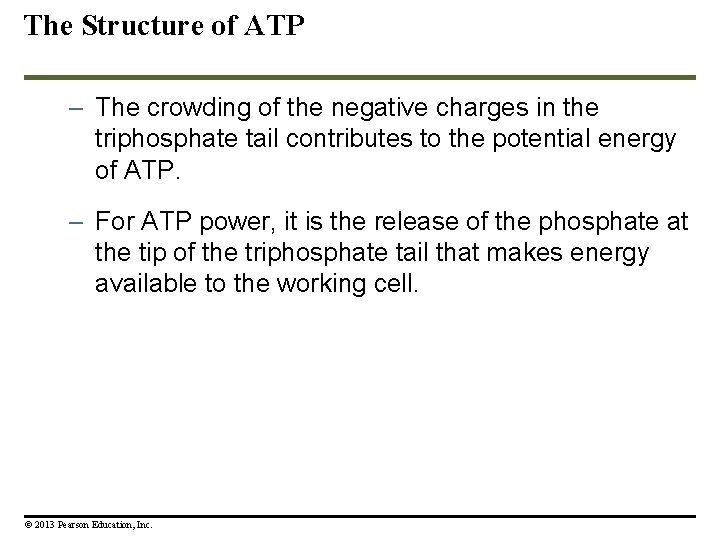
The Structure of ATP – The crowding of the negative charges in the triphosphate tail contributes to the potential energy of ATP. – For ATP power, it is the release of the phosphate at the tip of the triphosphate tail that makes energy available to the working cell. © 2013 Pearson Education, Inc.
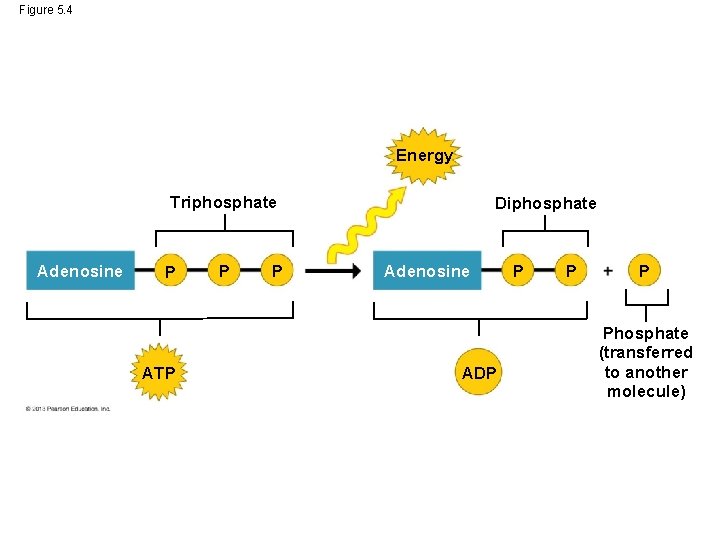
Figure 5. 4 Energy Triphosphate Adenosine P ATP P P Diphosphate Adenosine ADP P Phosphate (transferred to another molecule)
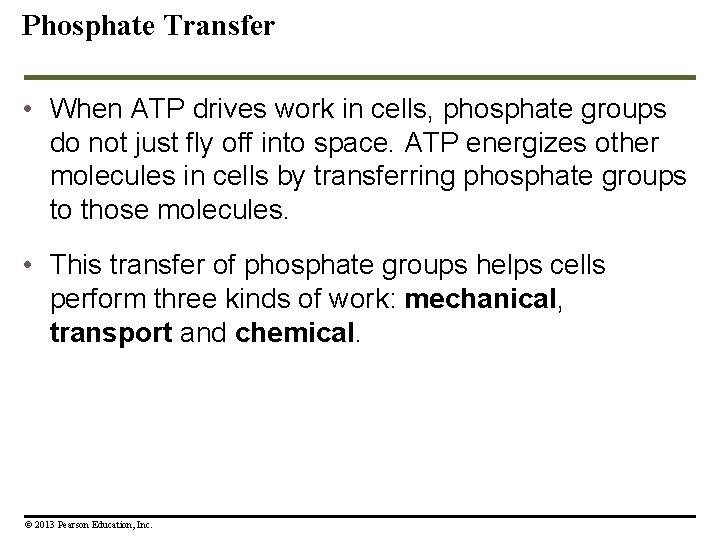
Phosphate Transfer • When ATP drives work in cells, phosphate groups do not just fly off into space. ATP energizes other molecules in cells by transferring phosphate groups to those molecules. • This transfer of phosphate groups helps cells perform three kinds of work: mechanical, transport and chemical. © 2013 Pearson Education, Inc.
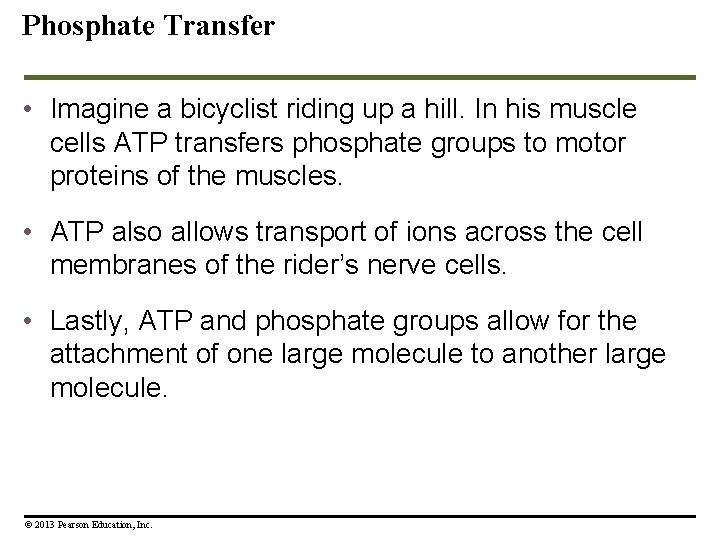
Phosphate Transfer • Imagine a bicyclist riding up a hill. In his muscle cells ATP transfers phosphate groups to motor proteins of the muscles. • ATP also allows transport of ions across the cell membranes of the rider’s nerve cells. • Lastly, ATP and phosphate groups allow for the attachment of one large molecule to another large molecule. © 2013 Pearson Education, Inc.
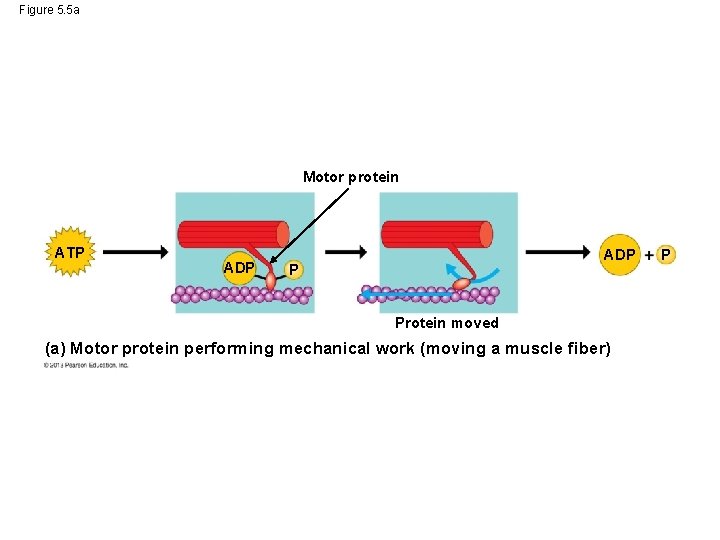
Figure 5. 5 a Motor protein ATP ADP P Protein moved (a) Motor protein performing mechanical work (moving a muscle fiber) P
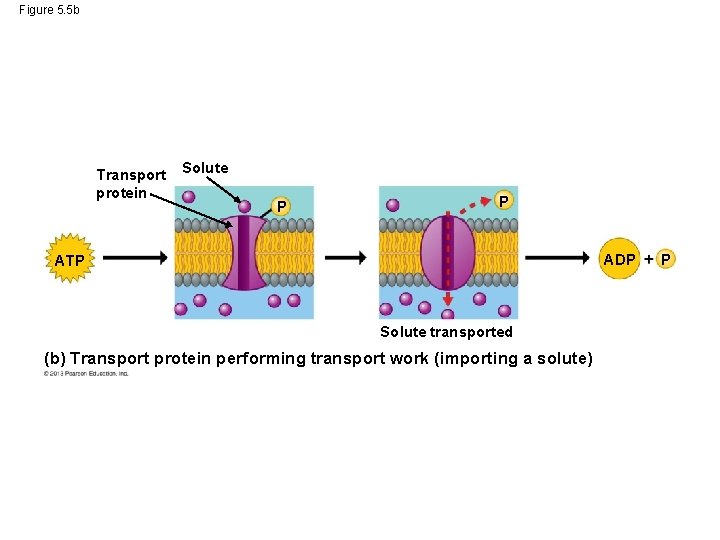
Figure 5. 5 b Transport protein Solute P P ADP ATP Solute transported (b) Transport protein performing transport work (importing a solute) P
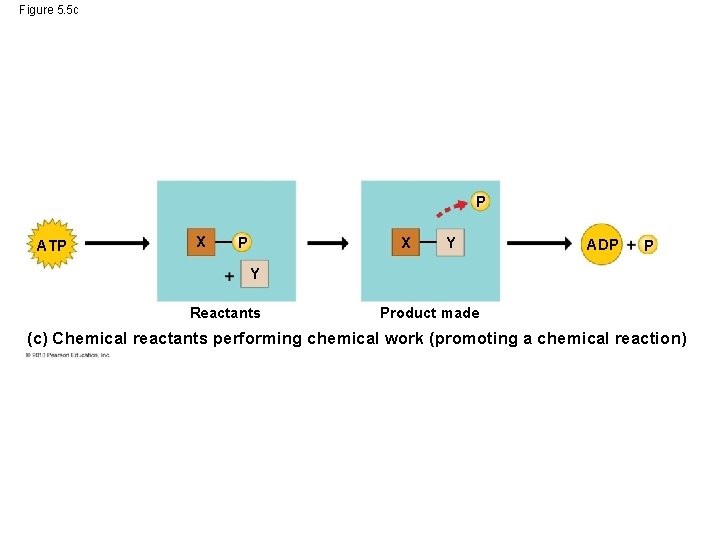
Figure 5. 5 c P ATP X Y ADP P Y Reactants Product made (c) Chemical reactants performing chemical work (promoting a chemical reaction)
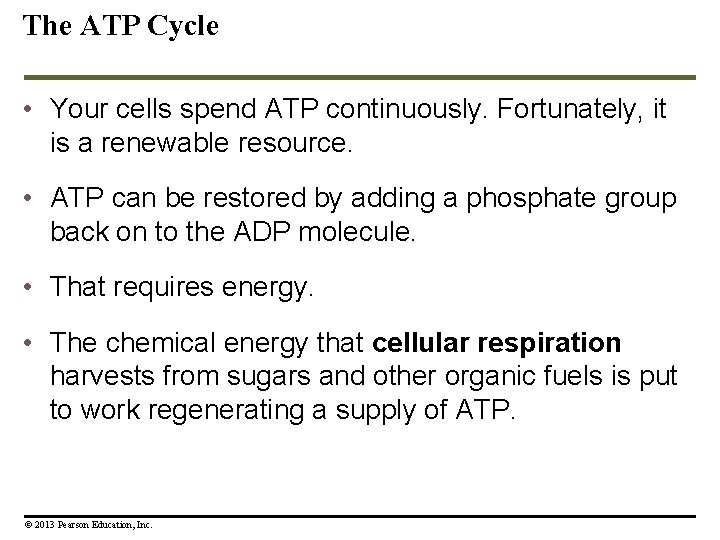
The ATP Cycle • Your cells spend ATP continuously. Fortunately, it is a renewable resource. • ATP can be restored by adding a phosphate group back on to the ADP molecule. • That requires energy. • The chemical energy that cellular respiration harvests from sugars and other organic fuels is put to work regenerating a supply of ATP. © 2013 Pearson Education, Inc.
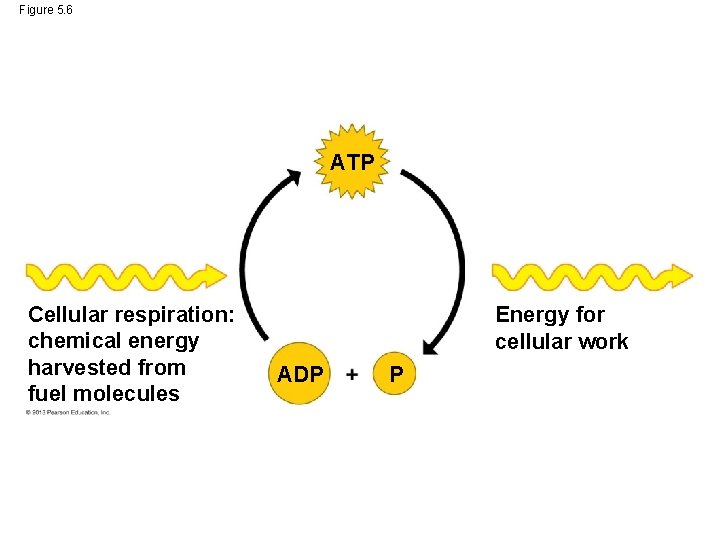
Figure 5. 6 ATP Cellular respiration: chemical energy harvested from fuel molecules Energy for cellular work ADP P
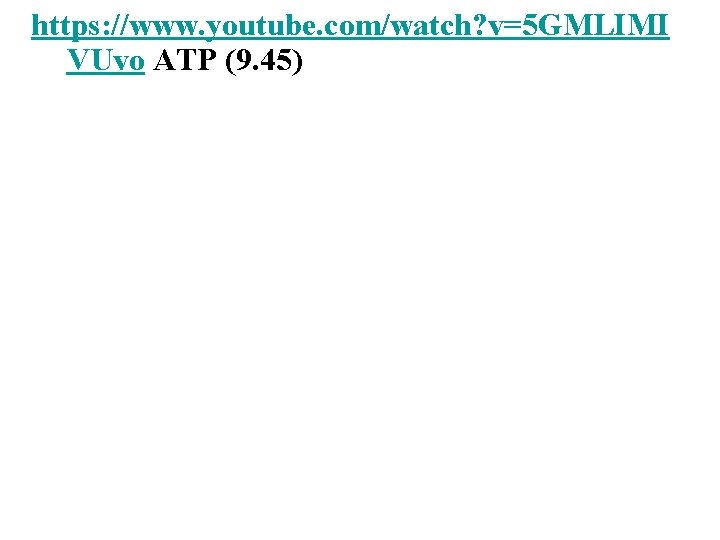
https: //www. youtube. com/watch? v=5 GMLIMI VUvo ATP (9. 45)
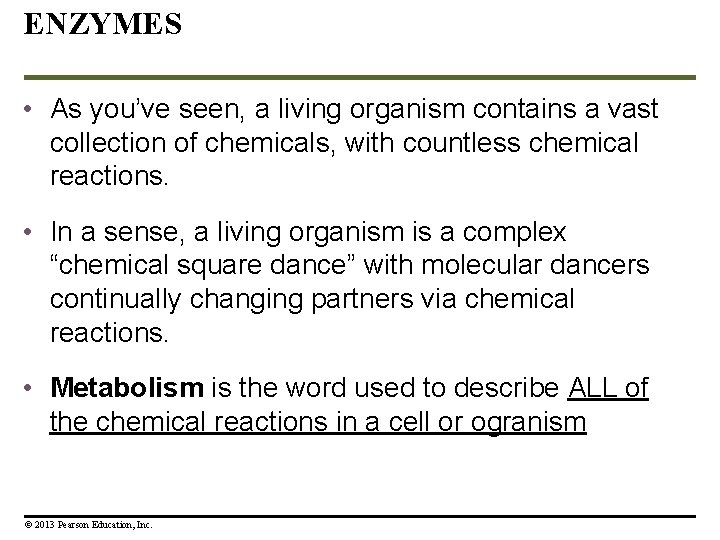
ENZYMES • As you’ve seen, a living organism contains a vast collection of chemicals, with countless chemical reactions. • In a sense, a living organism is a complex “chemical square dance” with molecular dancers continually changing partners via chemical reactions. • Metabolism is the word used to describe ALL of the chemical reactions in a cell or ogranism © 2013 Pearson Education, Inc.
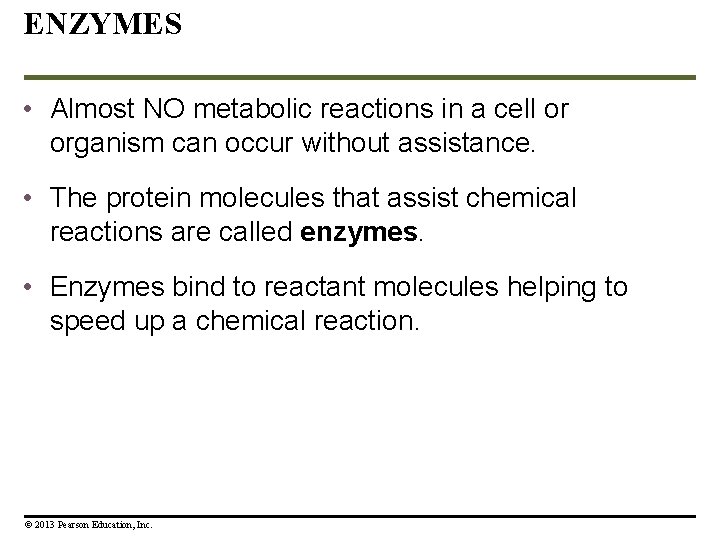
ENZYMES • Almost NO metabolic reactions in a cell or organism can occur without assistance. • The protein molecules that assist chemical reactions are called enzymes. • Enzymes bind to reactant molecules helping to speed up a chemical reaction. © 2013 Pearson Education, Inc.
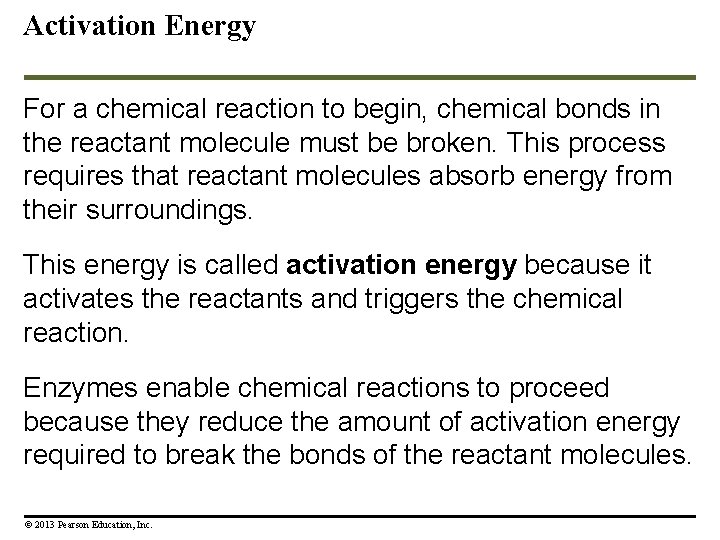
Activation Energy For a chemical reaction to begin, chemical bonds in the reactant molecule must be broken. This process requires that reactant molecules absorb energy from their surroundings. This energy is called activation energy because it activates the reactants and triggers the chemical reaction. Enzymes enable chemical reactions to proceed because they reduce the amount of activation energy required to break the bonds of the reactant molecules. © 2013 Pearson Education, Inc.
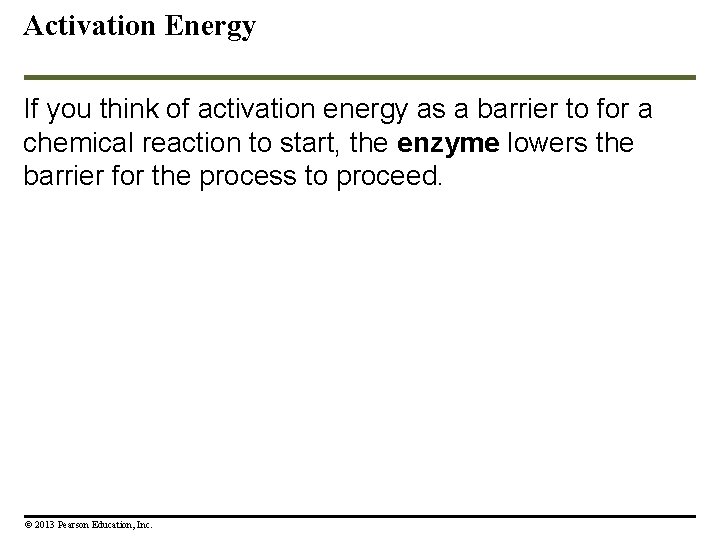
Activation Energy If you think of activation energy as a barrier to for a chemical reaction to start, the enzyme lowers the barrier for the process to proceed. © 2013 Pearson Education, Inc.
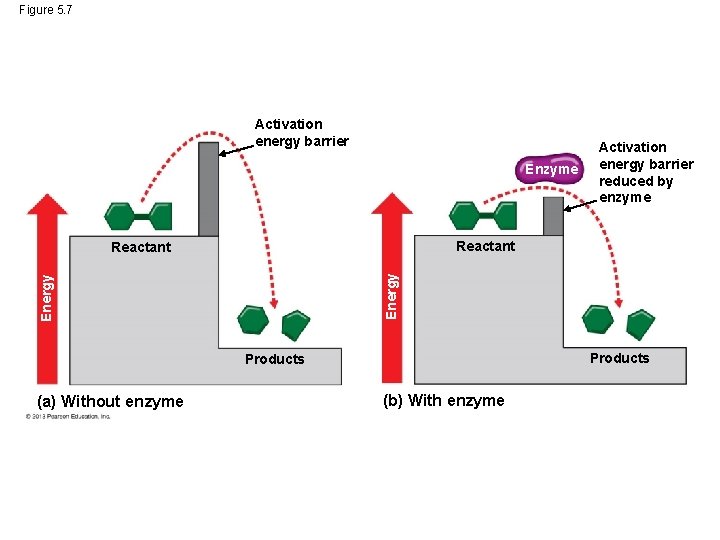
Figure 5. 7 Activation energy barrier Enzyme Reactant Energy Reactant Products (a) Without enzyme Activation energy barrier reduced by enzyme (b) With enzyme
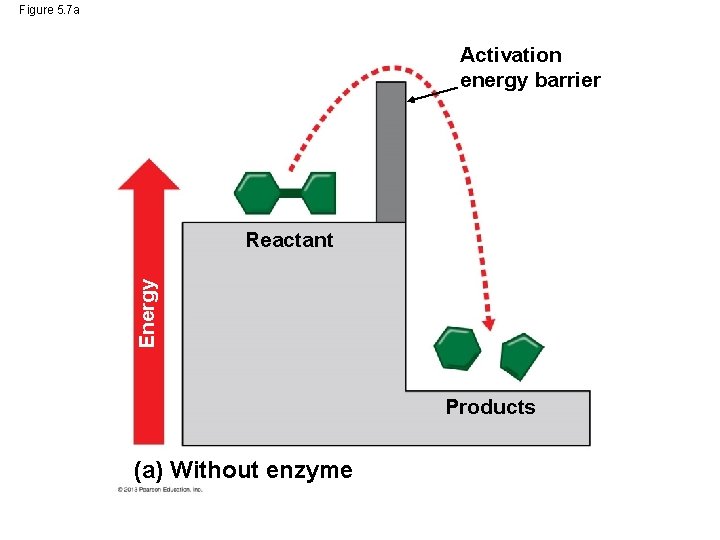
Figure 5. 7 a Activation energy barrier Energy Reactant Products (a) Without enzyme
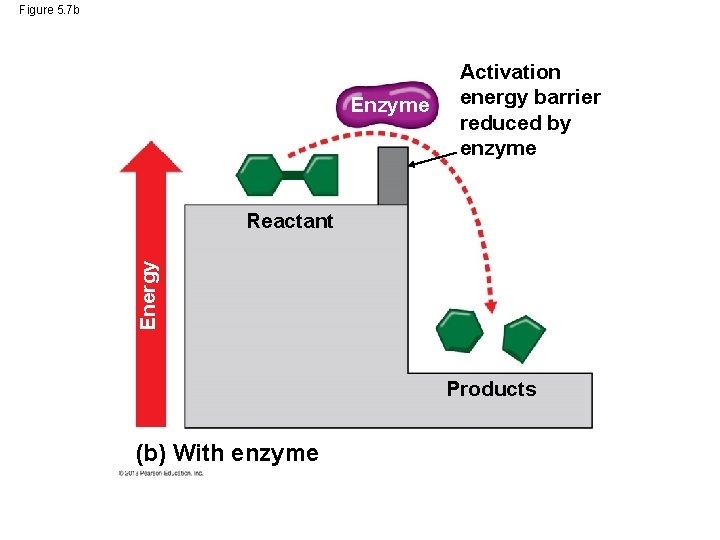
Figure 5. 7 b Enzyme Activation energy barrier reduced by enzyme Energy Reactant Products (b) With enzyme
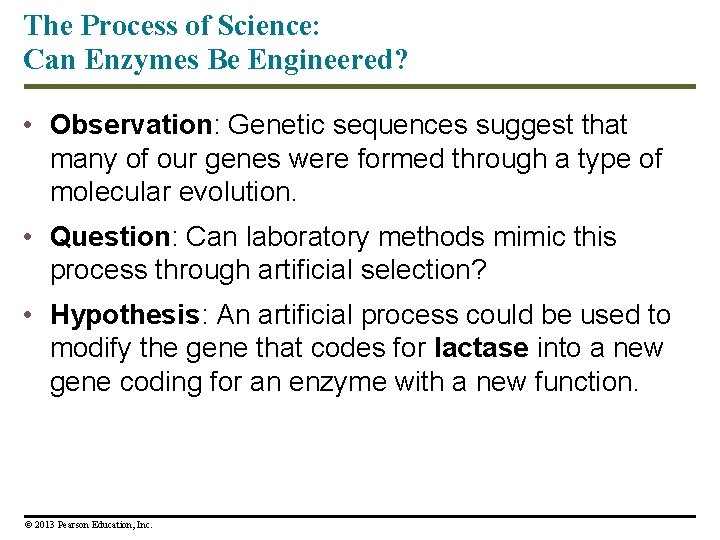
The Process of Science: Can Enzymes Be Engineered? • Observation: Genetic sequences suggest that many of our genes were formed through a type of molecular evolution. • Question: Can laboratory methods mimic this process through artificial selection? • Hypothesis: An artificial process could be used to modify the gene that codes for lactase into a new gene coding for an enzyme with a new function. © 2013 Pearson Education, Inc.
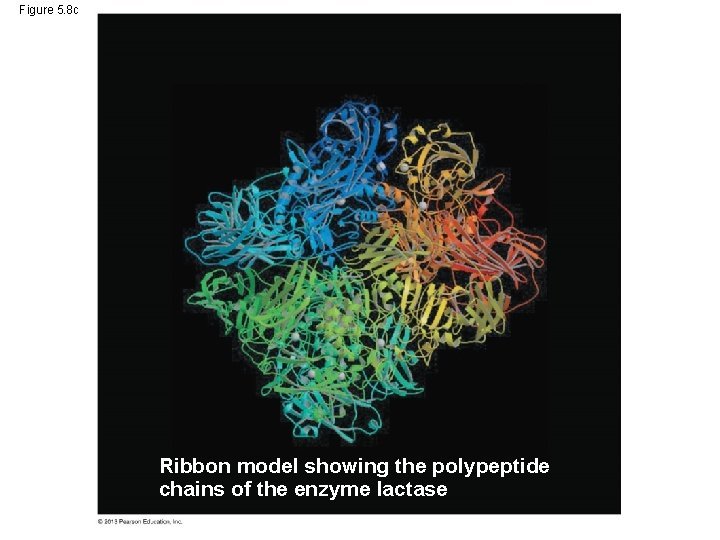
Figure 5. 8 c Ribbon model showing the polypeptide chains of the enzyme lactase
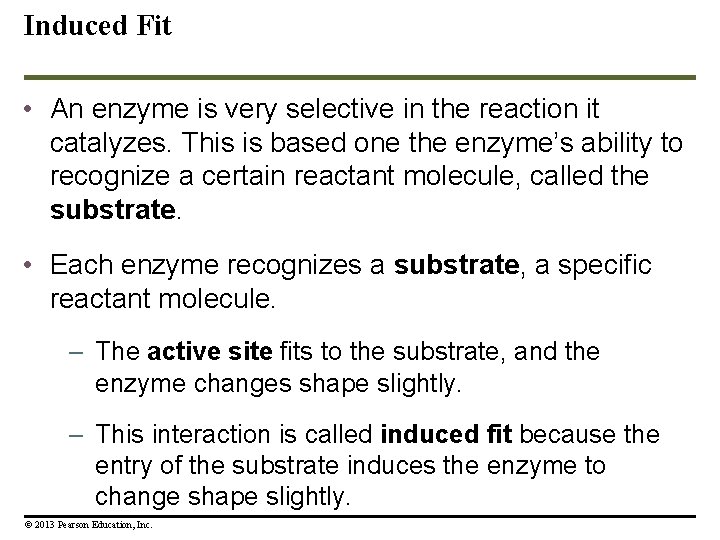
Induced Fit • An enzyme is very selective in the reaction it catalyzes. This is based one the enzyme’s ability to recognize a certain reactant molecule, called the substrate. • Each enzyme recognizes a substrate, a specific reactant molecule. – The active site fits to the substrate, and the enzyme changes shape slightly. – This interaction is called induced fit because the entry of the substrate induces the enzyme to change shape slightly. © 2013 Pearson Education, Inc.
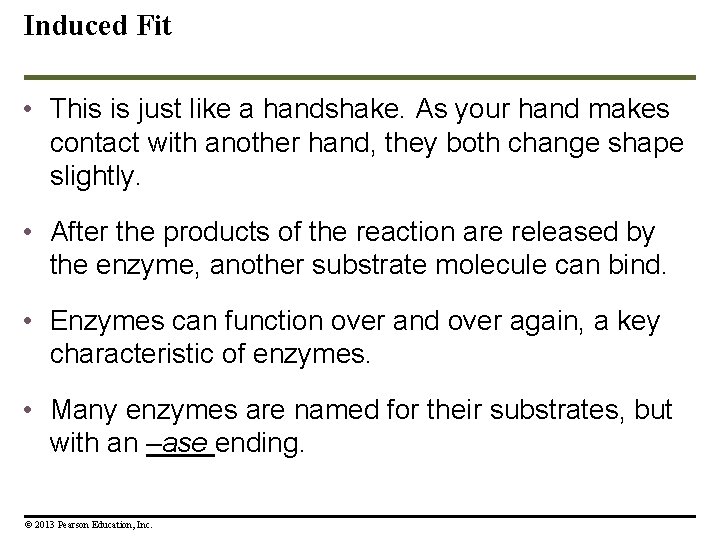
Induced Fit • This is just like a handshake. As your hand makes contact with another hand, they both change shape slightly. • After the products of the reaction are released by the enzyme, another substrate molecule can bind. • Enzymes can function over and over again, a key characteristic of enzymes. • Many enzymes are named for their substrates, but with an –ase ending. © 2013 Pearson Education, Inc.
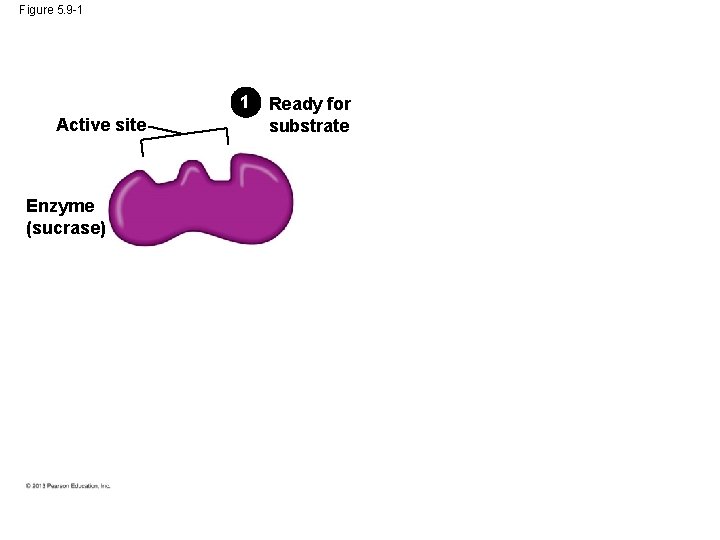
Figure 5. 9 -1 1 Active site Enzyme (sucrase) Ready for substrate
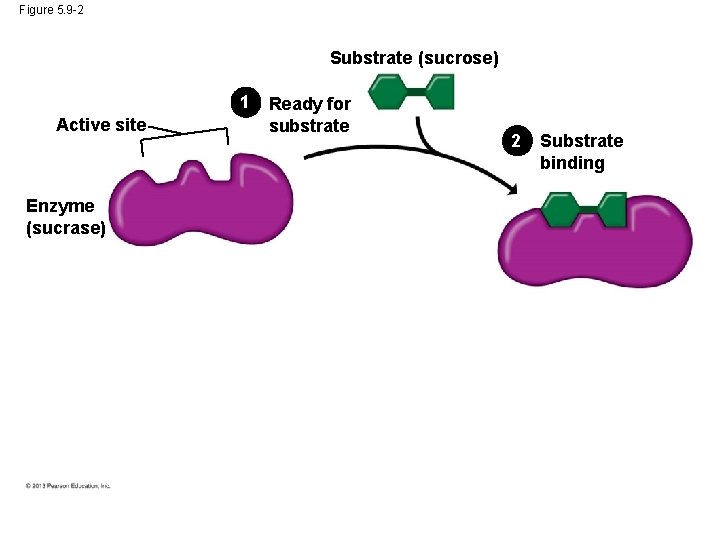
Figure 5. 9 -2 Substrate (sucrose) 1 Active site Enzyme (sucrase) Ready for substrate 2 Substrate binding
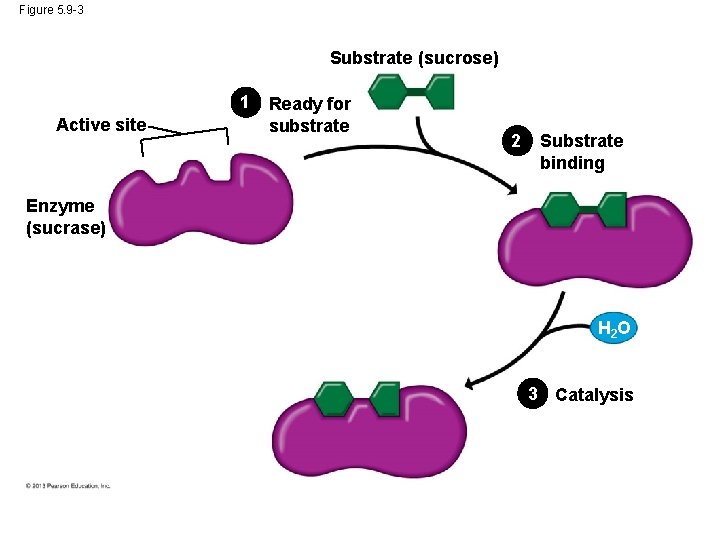
Figure 5. 9 -3 Substrate (sucrose) 1 Active site Ready for substrate 2 Substrate binding Enzyme (sucrase) H 2 O 3 Catalysis
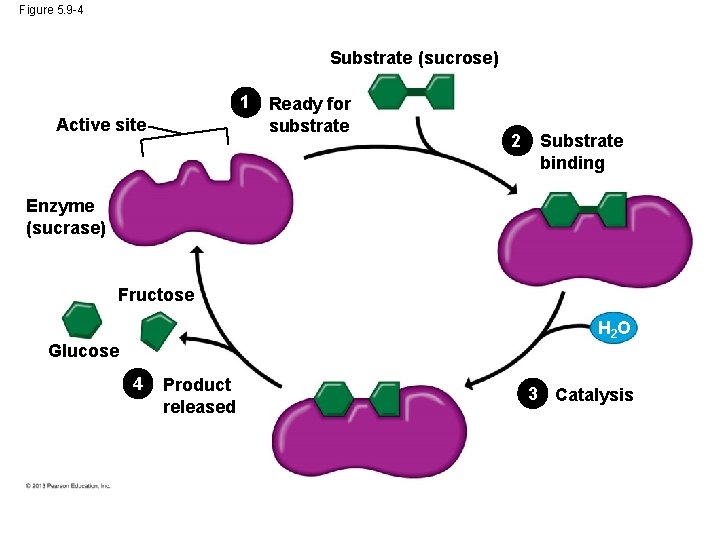
Figure 5. 9 -4 Substrate (sucrose) 1 Active site Ready for substrate 2 Substrate binding Enzyme (sucrase) Fructose H 2 O Glucose 4 Product released 3 Catalysis
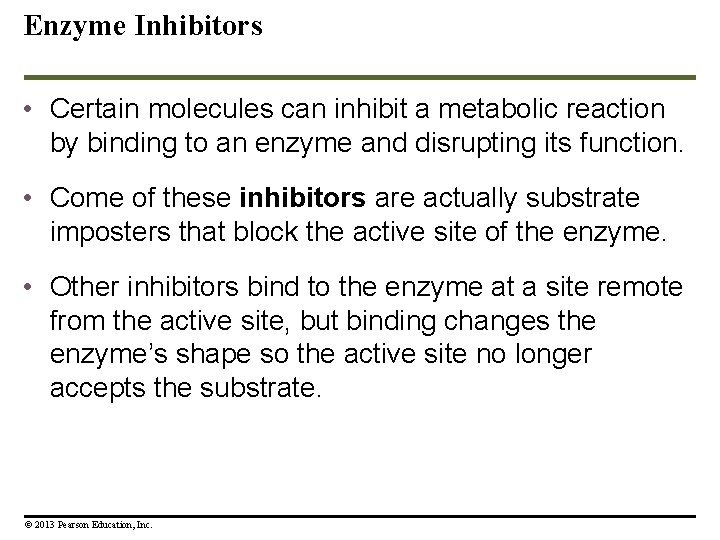
Enzyme Inhibitors • Certain molecules can inhibit a metabolic reaction by binding to an enzyme and disrupting its function. • Come of these inhibitors are actually substrate imposters that block the active site of the enzyme. • Other inhibitors bind to the enzyme at a site remote from the active site, but binding changes the enzyme’s shape so the active site no longer accepts the substrate. © 2013 Pearson Education, Inc.
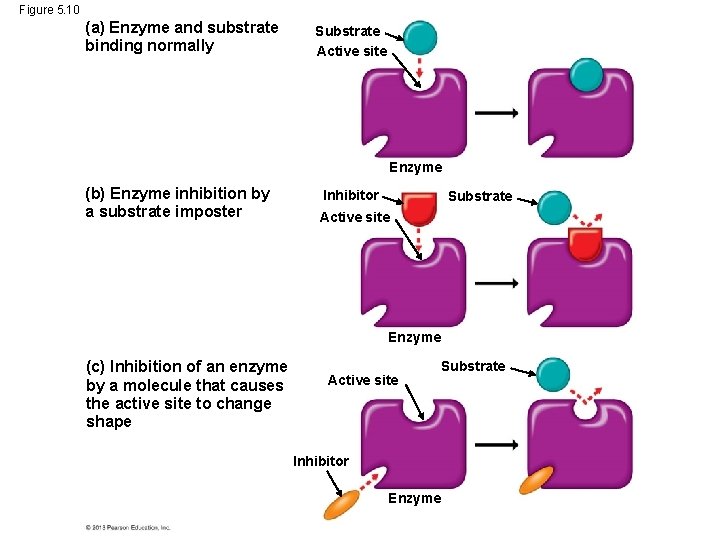
Figure 5. 10 (a) Enzyme and substrate binding normally Substrate Active site Enzyme (b) Enzyme inhibition by a substrate imposter Inhibitor Substrate Active site Enzyme (c) Inhibition of an enzyme by a molecule that causes the active site to change shape Active site Substrate Inhibitor Enzyme
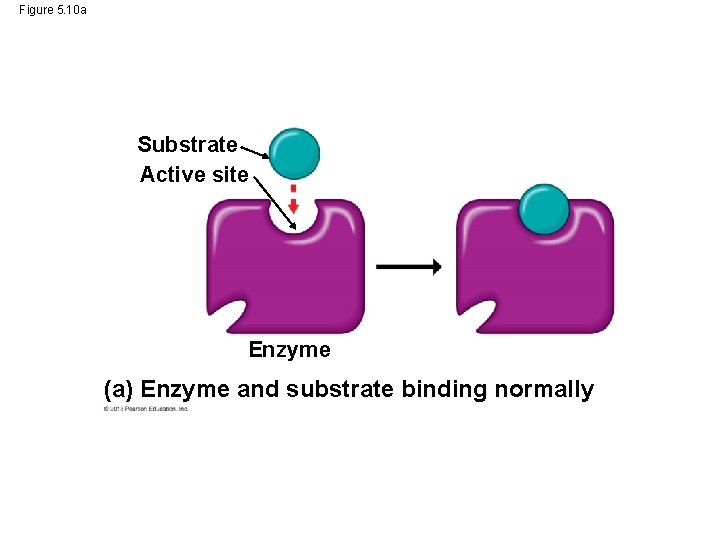
Figure 5. 10 a Substrate Active site Enzyme (a) Enzyme and substrate binding normally
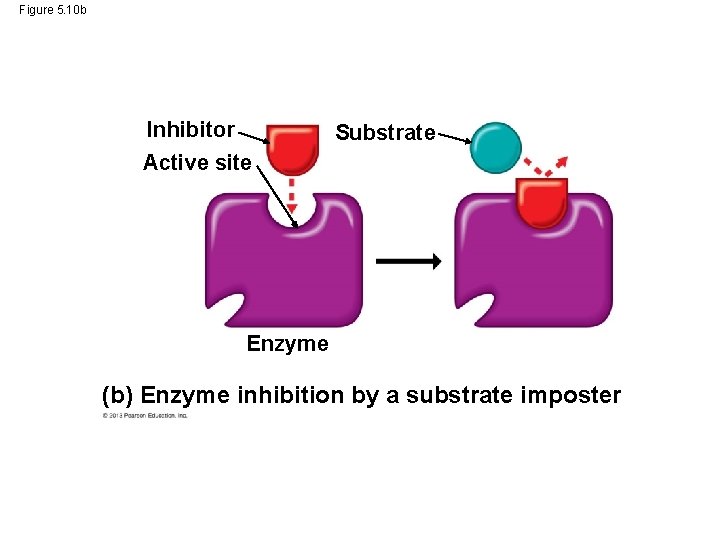
Figure 5. 10 b Inhibitor Active site Substrate Enzyme (b) Enzyme inhibition by a substrate imposter
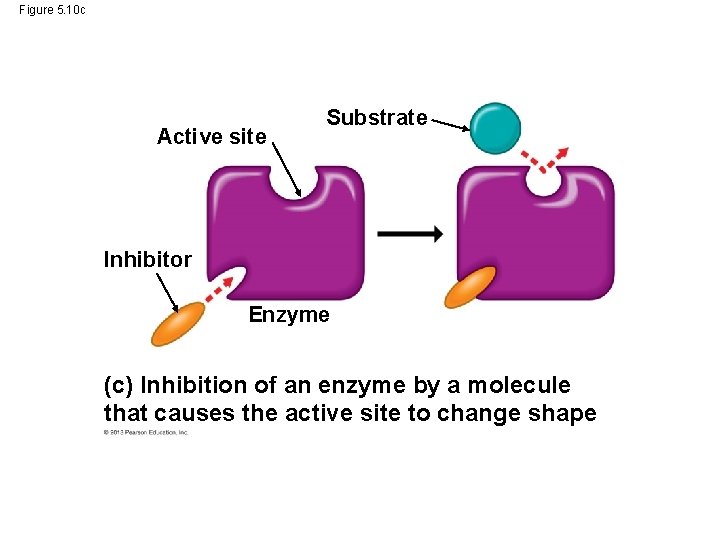
Figure 5. 10 c Active site Substrate Inhibitor Enzyme (c) Inhibition of an enzyme by a molecule that causes the active site to change shape
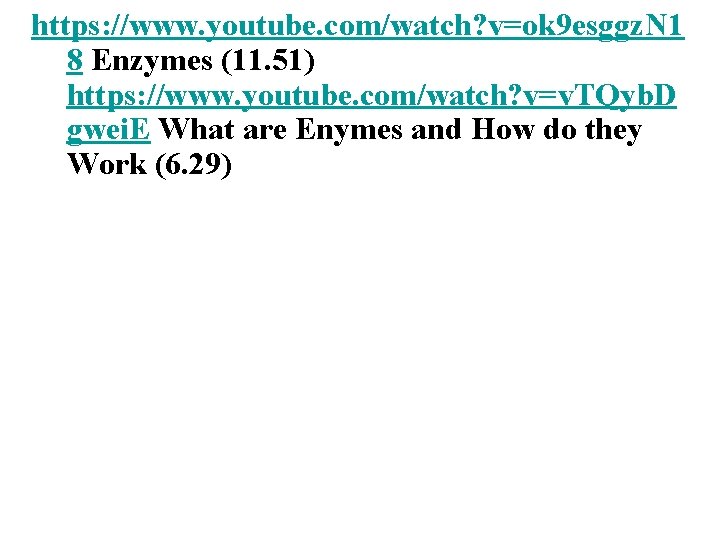
https: //www. youtube. com/watch? v=ok 9 esggz. N 1 8 Enzymes (11. 51) https: //www. youtube. com/watch? v=v. TQyb. D gwei. E What are Enymes and How do they Work (6. 29)
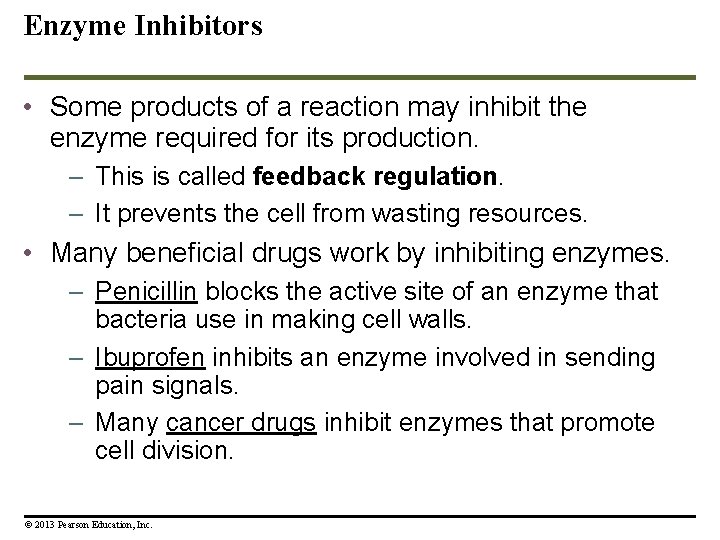
Enzyme Inhibitors • Some products of a reaction may inhibit the enzyme required for its production. – This is called feedback regulation. – It prevents the cell from wasting resources. • Many beneficial drugs work by inhibiting enzymes. – Penicillin blocks the active site of an enzyme that bacteria use in making cell walls. – Ibuprofen inhibits an enzyme involved in sending pain signals. – Many cancer drugs inhibit enzymes that promote cell division. © 2013 Pearson Education, Inc.
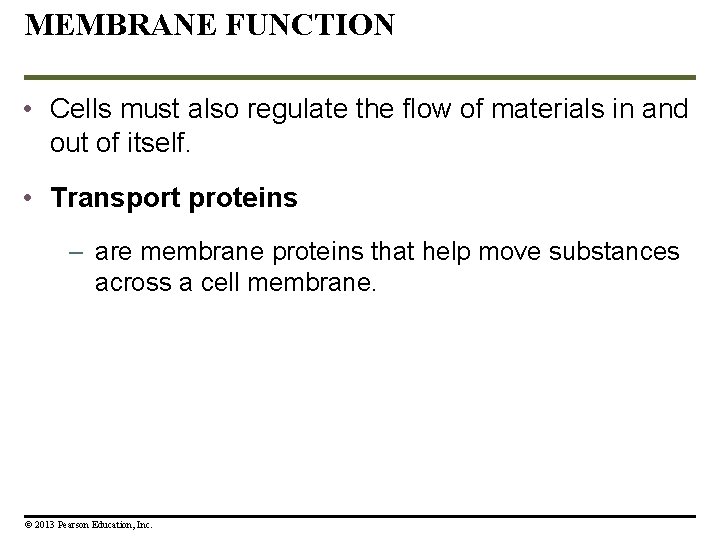
MEMBRANE FUNCTION • Cells must also regulate the flow of materials in and out of itself. • Transport proteins – are membrane proteins that help move substances across a cell membrane. © 2013 Pearson Education, Inc.
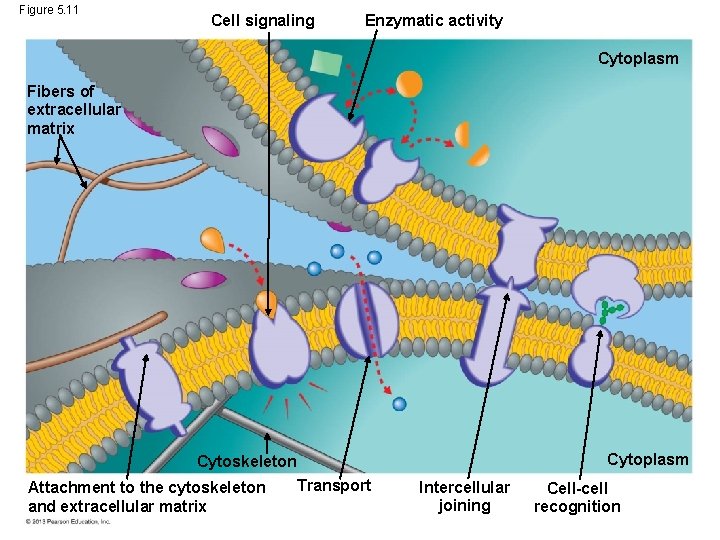
Figure 5. 11 Cell signaling Enzymatic activity Cytoplasm Fibers of extracellular matrix Cytoskeleton Transport Attachment to the cytoskeleton and extracellular matrix Cytoplasm Intercellular joining Cell-cell recognition
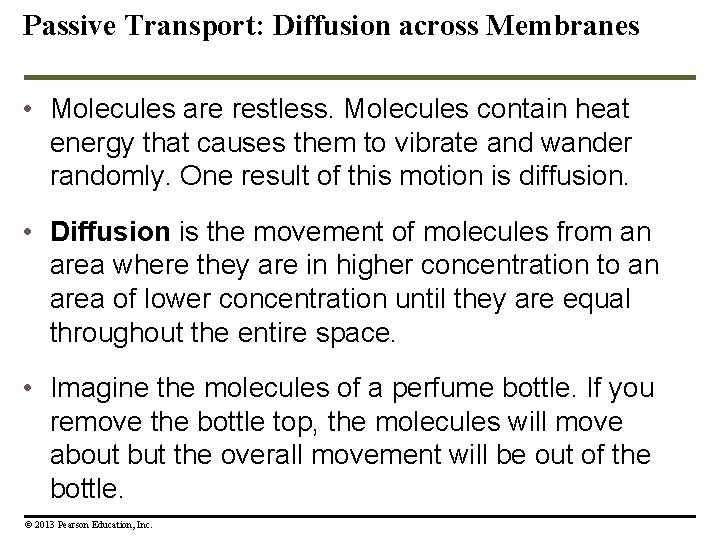
Passive Transport: Diffusion across Membranes • Molecules are restless. Molecules contain heat energy that causes them to vibrate and wander randomly. One result of this motion is diffusion. • Diffusion is the movement of molecules from an area where they are in higher concentration to an area of lower concentration until they are equal throughout the entire space. • Imagine the molecules of a perfume bottle. If you remove the bottle top, the molecules will move about but the overall movement will be out of the bottle. © 2013 Pearson Education, Inc.
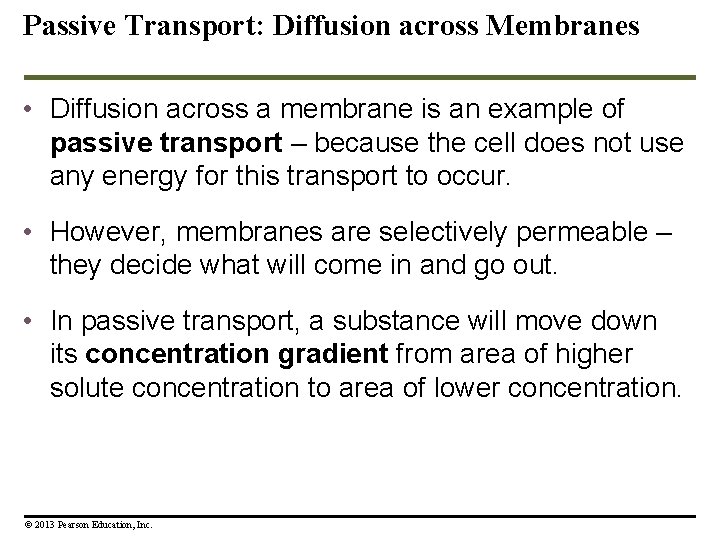
Passive Transport: Diffusion across Membranes • Diffusion across a membrane is an example of passive transport – because the cell does not use any energy for this transport to occur. • However, membranes are selectively permeable – they decide what will come in and go out. • In passive transport, a substance will move down its concentration gradient from area of higher solute concentration to area of lower concentration. © 2013 Pearson Education, Inc.
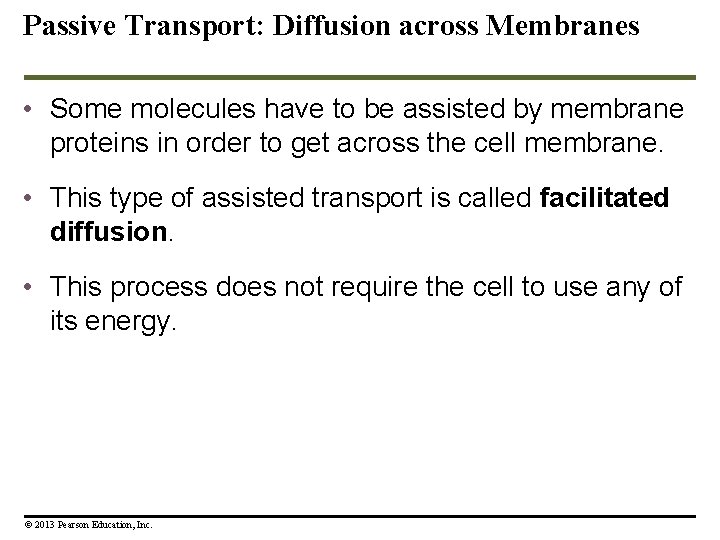
Passive Transport: Diffusion across Membranes • Some molecules have to be assisted by membrane proteins in order to get across the cell membrane. • This type of assisted transport is called facilitated diffusion. • This process does not require the cell to use any of its energy. © 2013 Pearson Education, Inc.
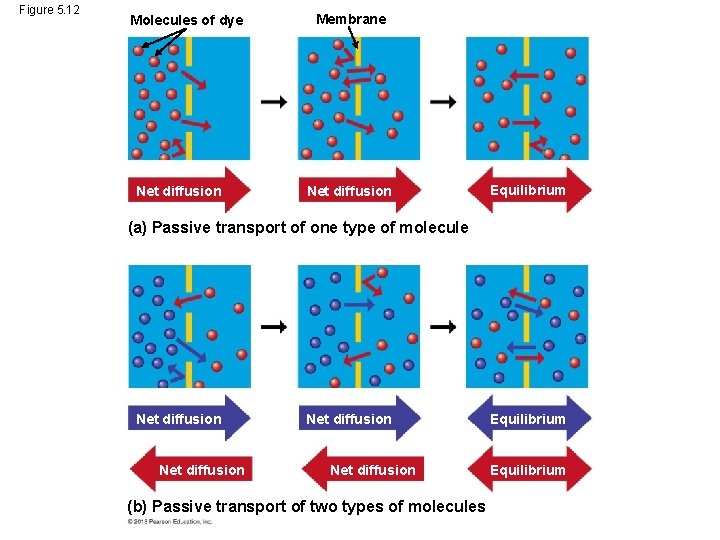
Figure 5. 12 Molecules of dye Net diffusion Membrane Net diffusion Equilibrium (a) Passive transport of one type of molecule Net diffusion (b) Passive transport of two types of molecules Equilibrium
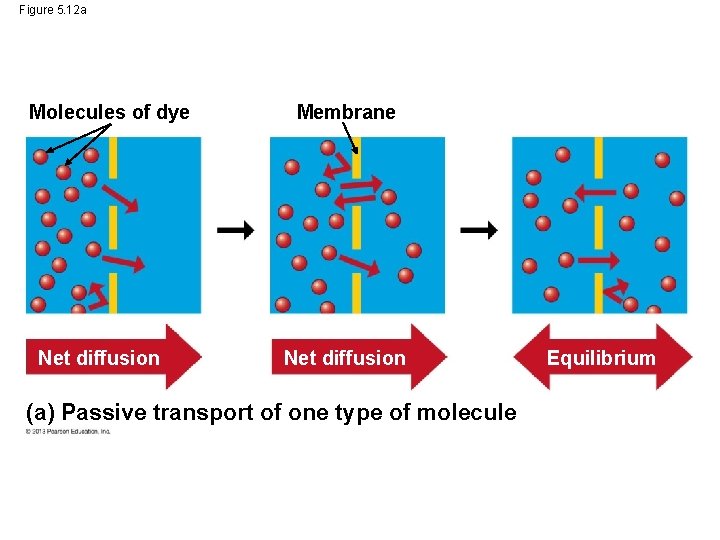
Figure 5. 12 a Molecules of dye Net diffusion Membrane Net diffusion (a) Passive transport of one type of molecule Equilibrium
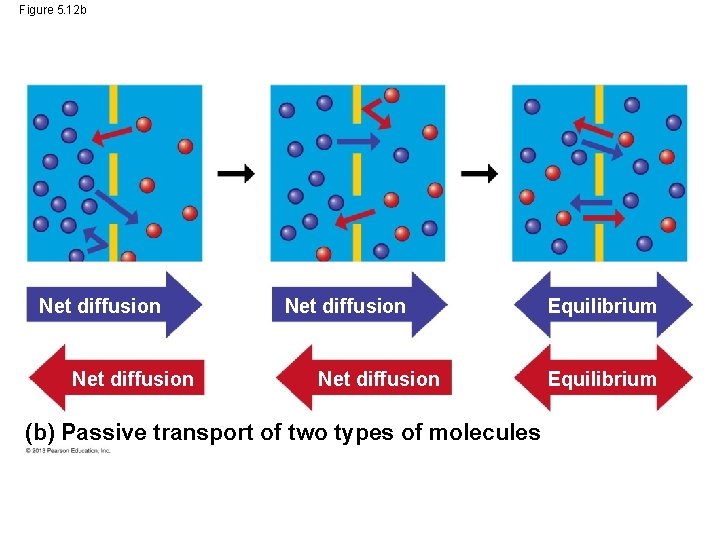
Figure 5. 12 b Net diffusion (b) Passive transport of two types of molecules Equilibrium
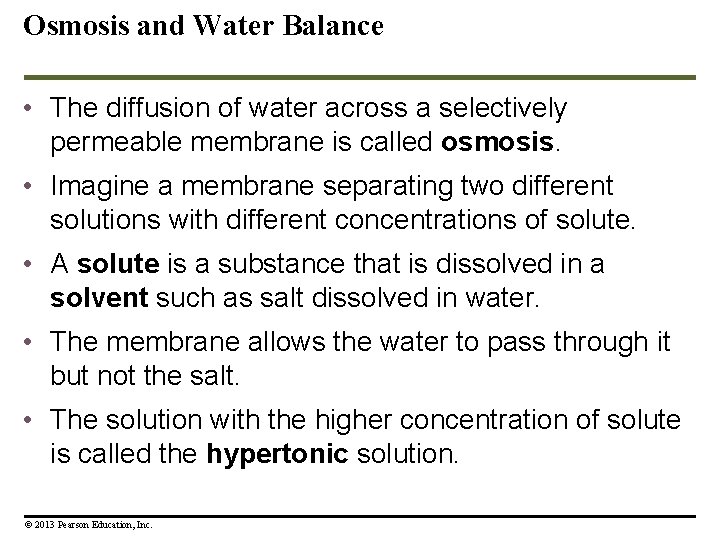
Osmosis and Water Balance • The diffusion of water across a selectively permeable membrane is called osmosis. • Imagine a membrane separating two different solutions with different concentrations of solute. • A solute is a substance that is dissolved in a solvent such as salt dissolved in water. • The membrane allows the water to pass through it but not the salt. • The solution with the higher concentration of solute is called the hypertonic solution. © 2013 Pearson Education, Inc.
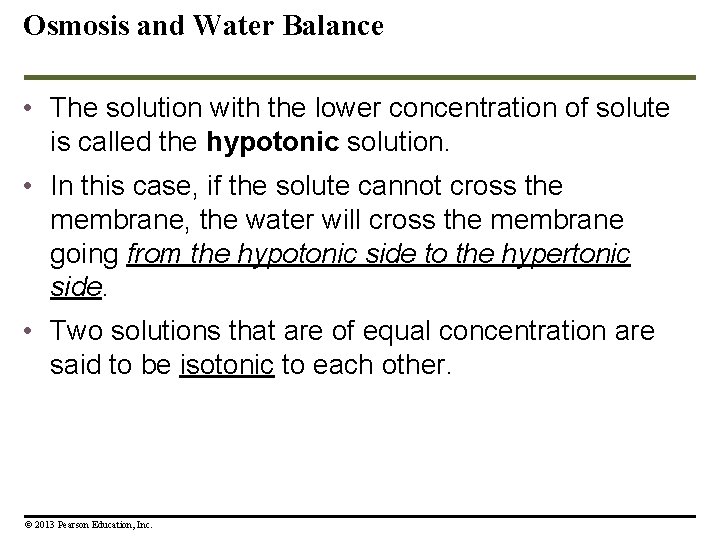
Osmosis and Water Balance • The solution with the lower concentration of solute is called the hypotonic solution. • In this case, if the solute cannot cross the membrane, the water will cross the membrane going from the hypotonic side to the hypertonic side. • Two solutions that are of equal concentration are said to be isotonic to each other. © 2013 Pearson Education, Inc.
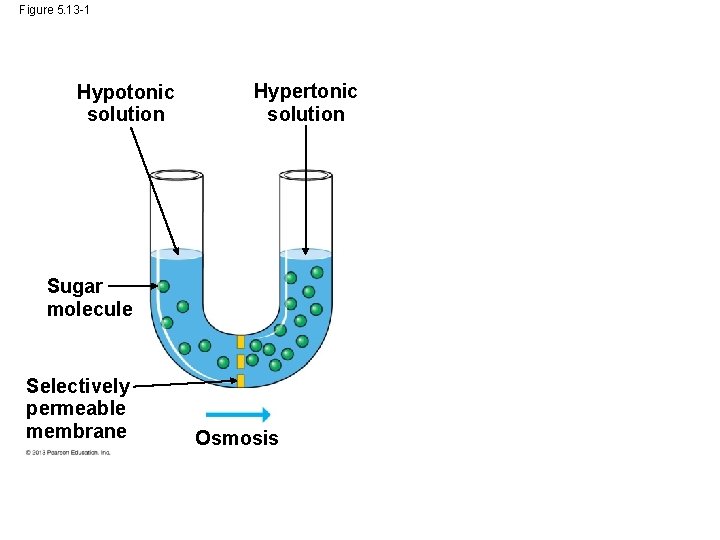
Figure 5. 13 -1 Hypotonic solution Hypertonic solution Sugar molecule Selectively permeable membrane Osmosis
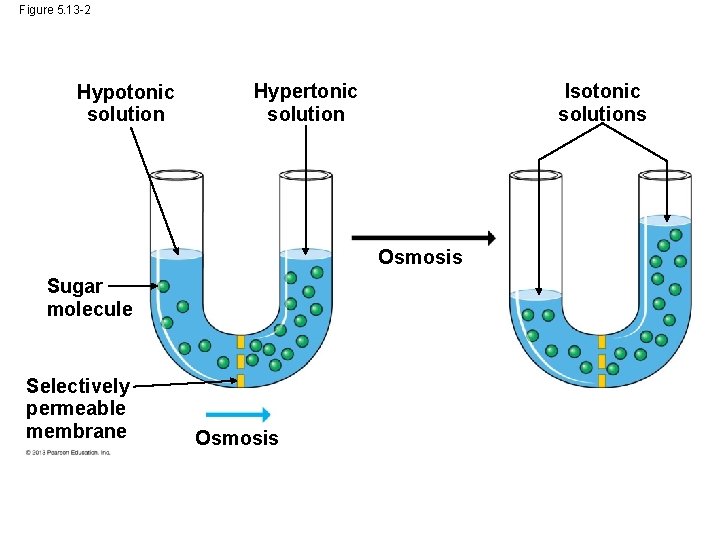
Figure 5. 13 -2 Hypotonic solution Isotonic solutions Hypertonic solution Osmosis Sugar molecule Selectively permeable membrane Osmosis
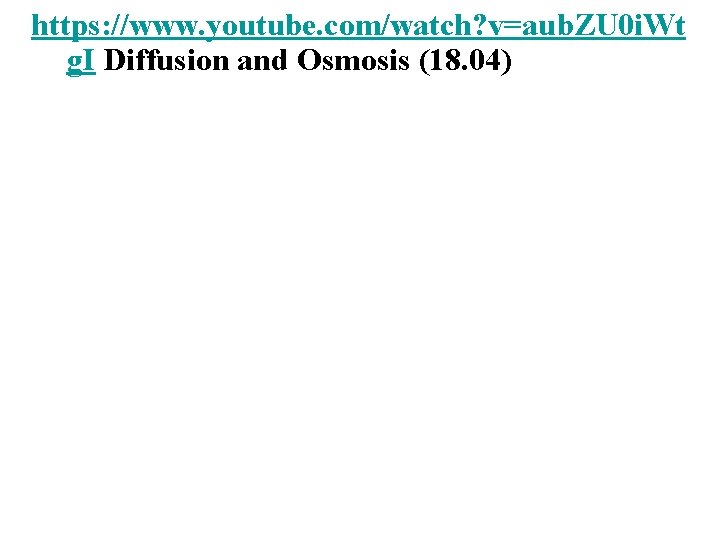
https: //www. youtube. com/watch? v=aub. ZU 0 i. Wt g. I Diffusion and Osmosis (18. 04)
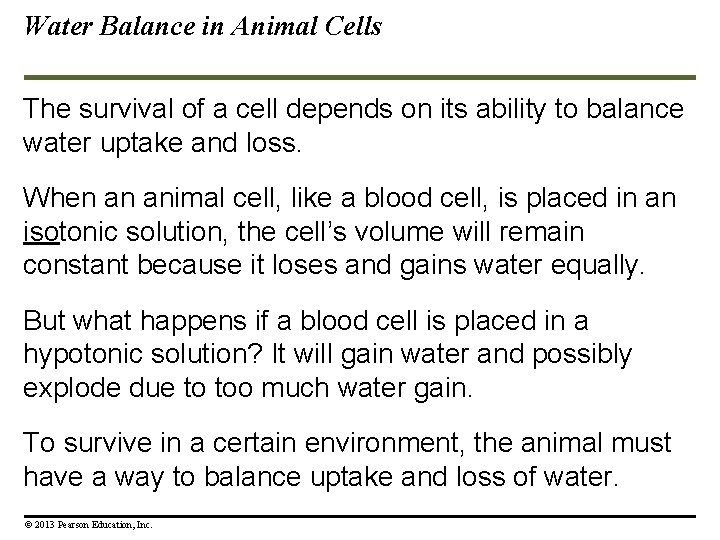
Water Balance in Animal Cells The survival of a cell depends on its ability to balance water uptake and loss. When an animal cell, like a blood cell, is placed in an isotonic solution, the cell’s volume will remain constant because it loses and gains water equally. But what happens if a blood cell is placed in a hypotonic solution? It will gain water and possibly explode due to too much water gain. To survive in a certain environment, the animal must have a way to balance uptake and loss of water. © 2013 Pearson Education, Inc.
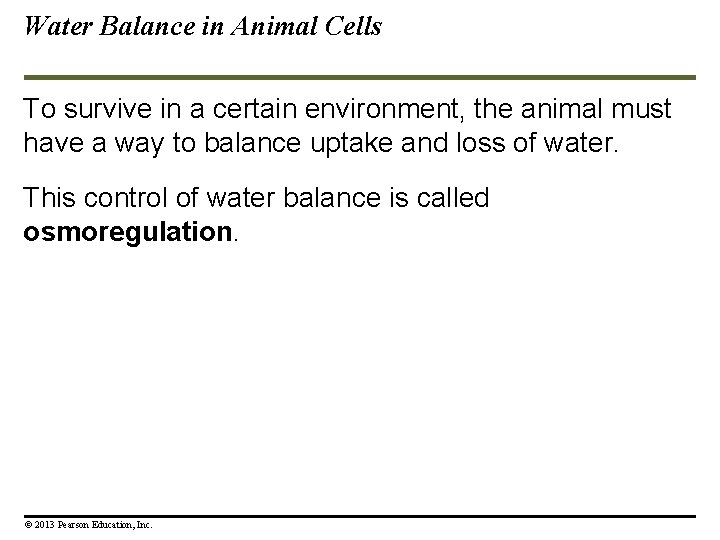
Water Balance in Animal Cells To survive in a certain environment, the animal must have a way to balance uptake and loss of water. This control of water balance is called osmoregulation. © 2013 Pearson Education, Inc.
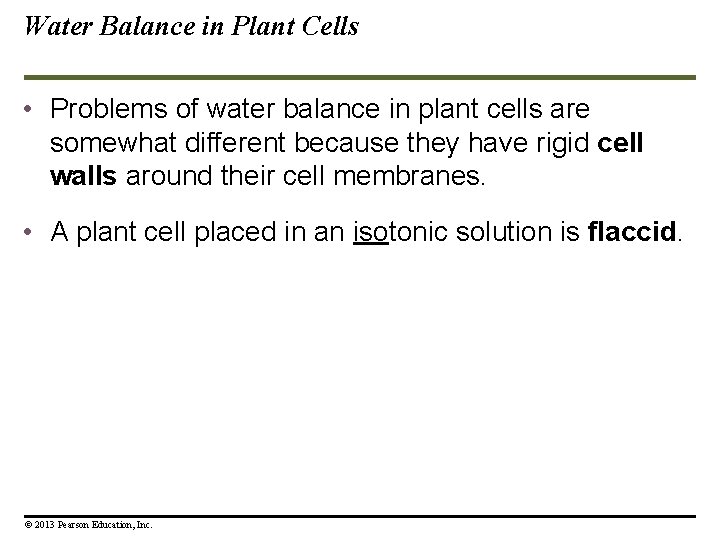
Water Balance in Plant Cells • Problems of water balance in plant cells are somewhat different because they have rigid cell walls around their cell membranes. • A plant cell placed in an isotonic solution is flaccid. © 2013 Pearson Education, Inc.
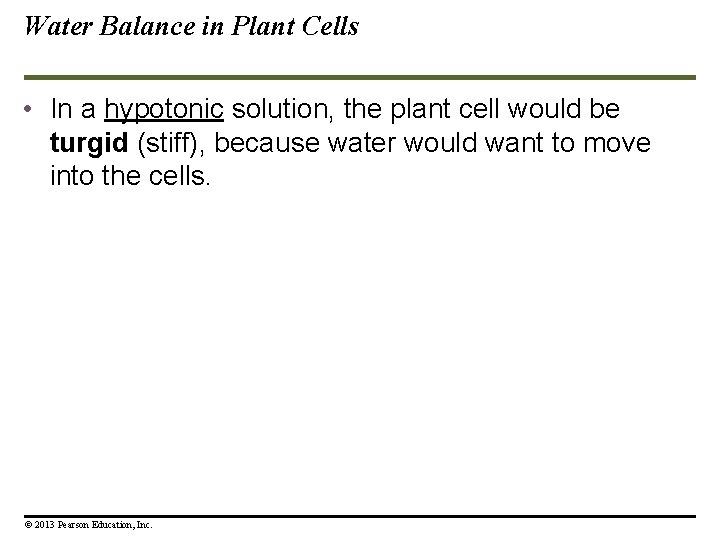
Water Balance in Plant Cells • In a hypotonic solution, the plant cell would be turgid (stiff), because water would want to move into the cells. © 2013 Pearson Education, Inc.
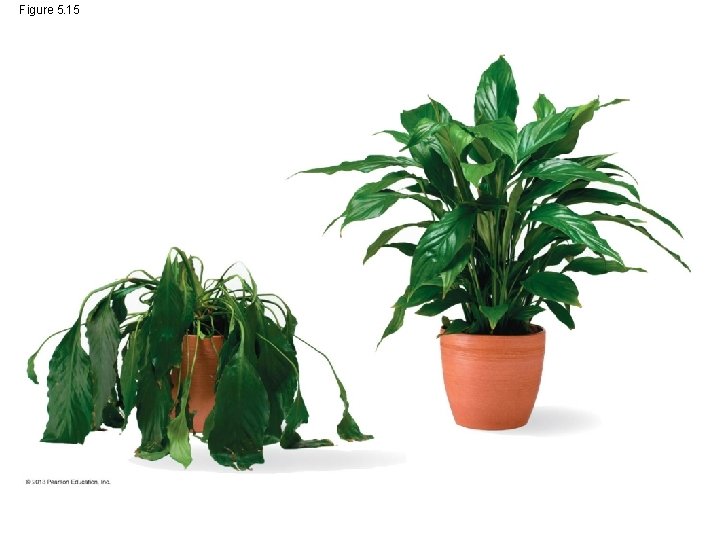
Figure 5. 15
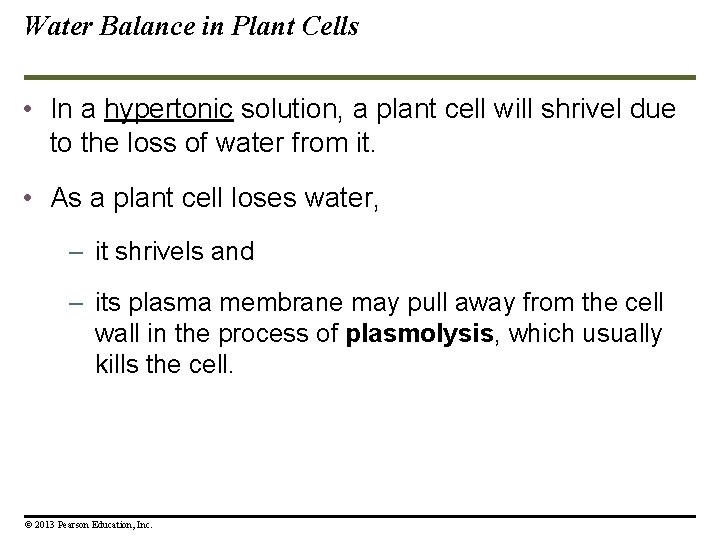
Water Balance in Plant Cells • In a hypertonic solution, a plant cell will shrivel due to the loss of water from it. • As a plant cell loses water, – it shrivels and – its plasma membrane may pull away from the cell wall in the process of plasmolysis, which usually kills the cell. © 2013 Pearson Education, Inc.
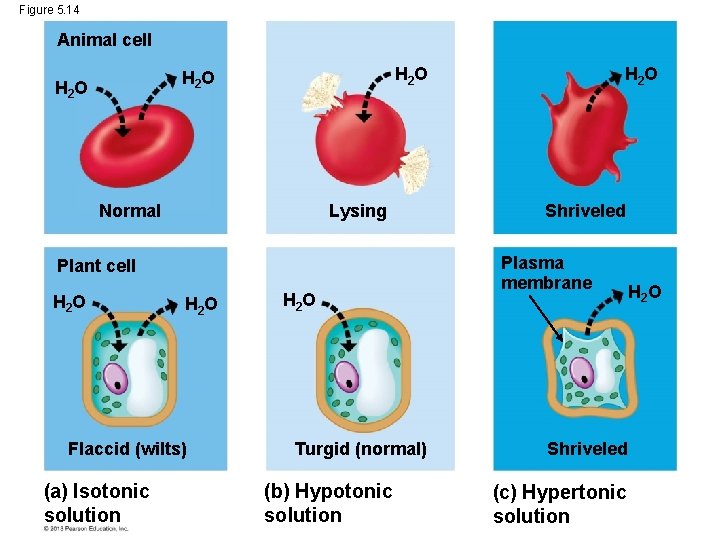
Figure 5. 14 Animal cell H 2 O Normal Lysing Plant cell H 2 O Flaccid (wilts) (a) Isotonic solution H 2 O Turgid (normal) (b) Hypotonic solution H 2 O Shriveled Plasma membrane Shriveled (c) Hypertonic solution H 2 O
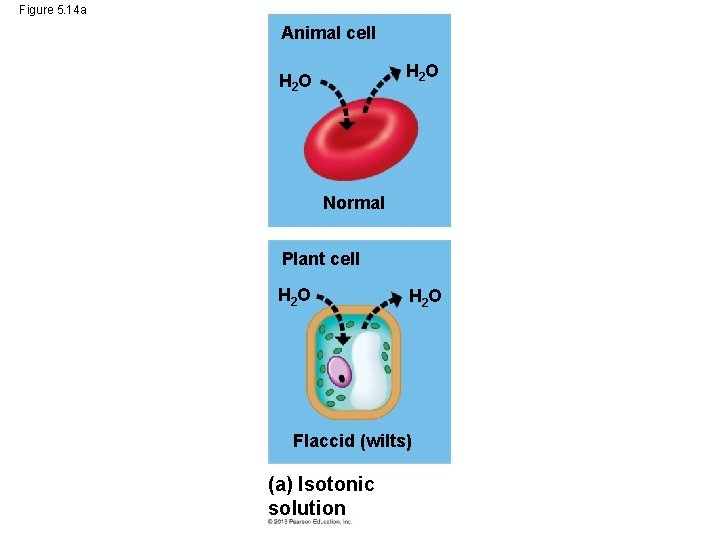
Figure 5. 14 a Animal cell H 2 O Normal Plant cell H 2 O Flaccid (wilts) (a) Isotonic solution
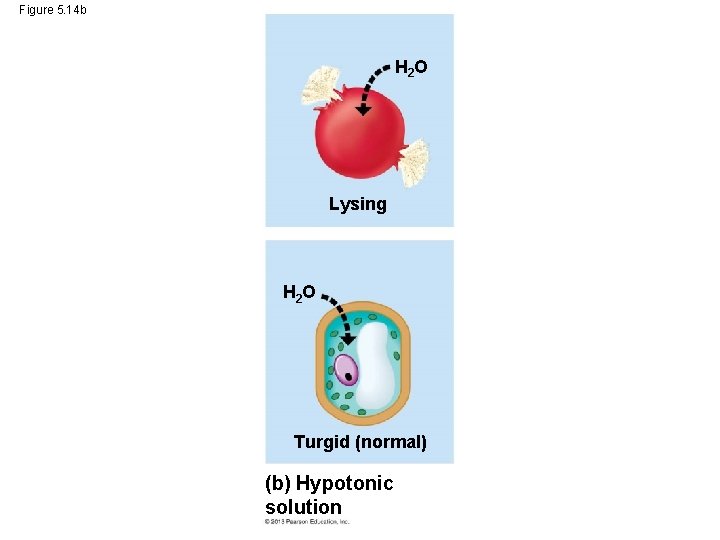
Figure 5. 14 b H 2 O Lysing H 2 O Turgid (normal) (b) Hypotonic solution
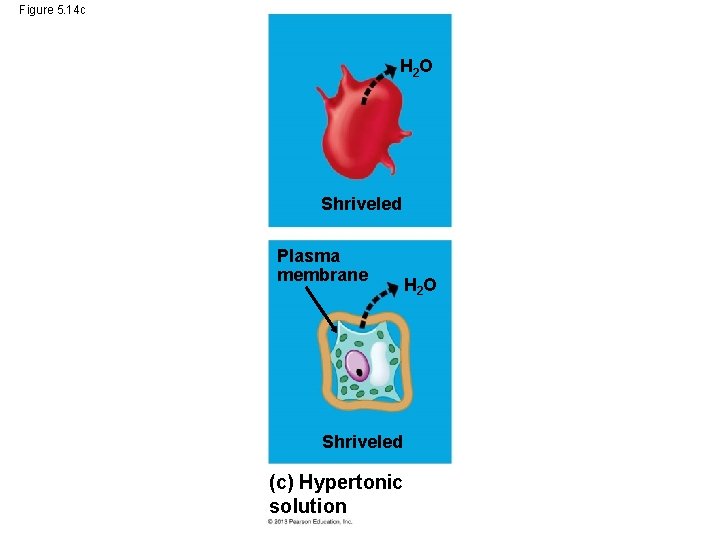
Figure 5. 14 c H 2 O Shriveled Plasma membrane H 2 O Shriveled (c) Hypertonic solution
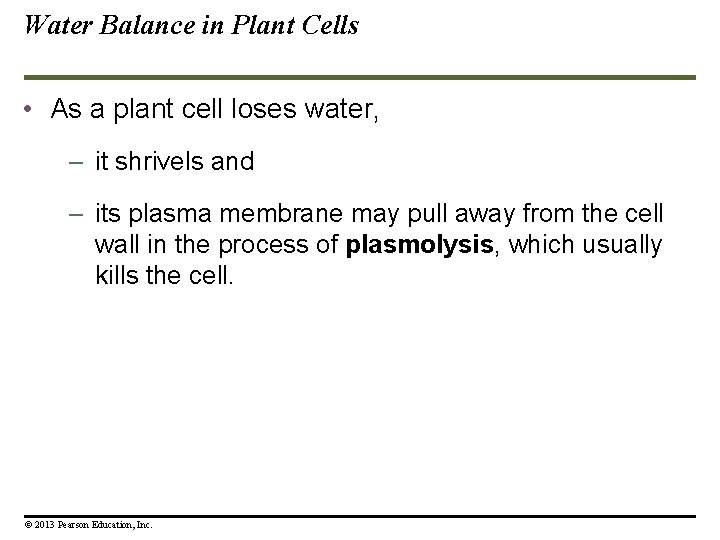
Water Balance in Plant Cells • As a plant cell loses water, – it shrivels and – its plasma membrane may pull away from the cell wall in the process of plasmolysis, which usually kills the cell. © 2013 Pearson Education, Inc.
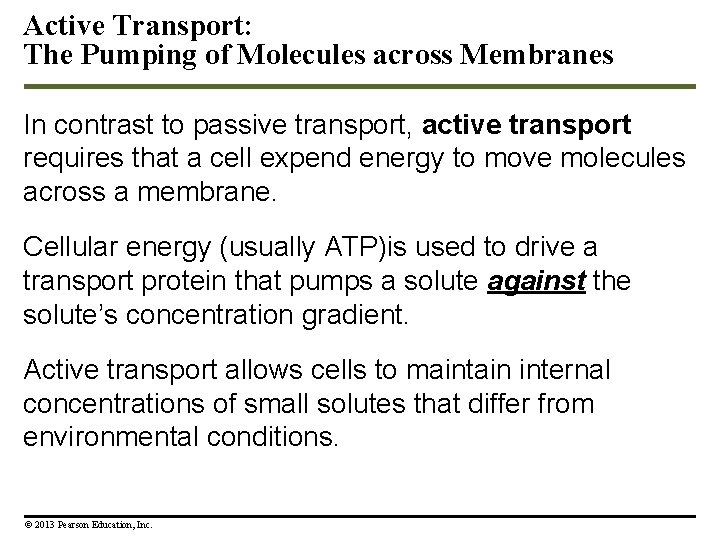
Active Transport: The Pumping of Molecules across Membranes In contrast to passive transport, active transport requires that a cell expend energy to move molecules across a membrane. Cellular energy (usually ATP)is used to drive a transport protein that pumps a solute against the solute’s concentration gradient. Active transport allows cells to maintain internal concentrations of small solutes that differ from environmental conditions. © 2013 Pearson Education, Inc.
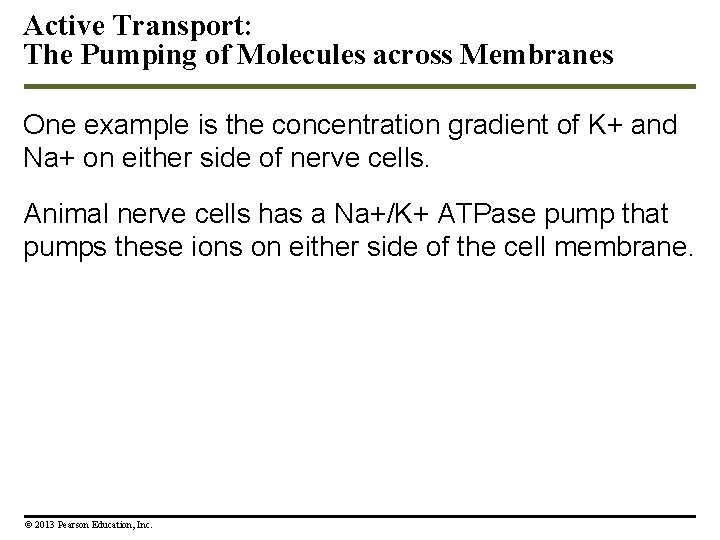
Active Transport: The Pumping of Molecules across Membranes One example is the concentration gradient of K+ and Na+ on either side of nerve cells. Animal nerve cells has a Na+/K+ ATPase pump that pumps these ions on either side of the cell membrane. © 2013 Pearson Education, Inc.
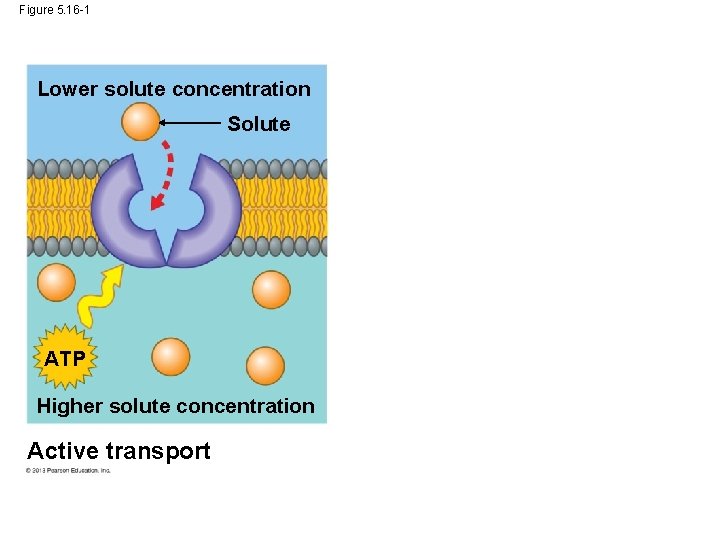
Figure 5. 16 -1 Lower solute concentration Solute ATP Higher solute concentration Active transport
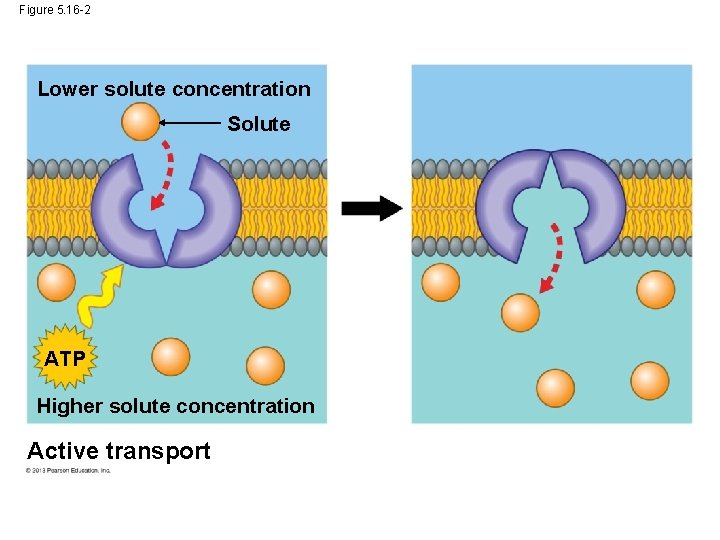
Figure 5. 16 -2 Lower solute concentration Solute ATP Higher solute concentration Active transport
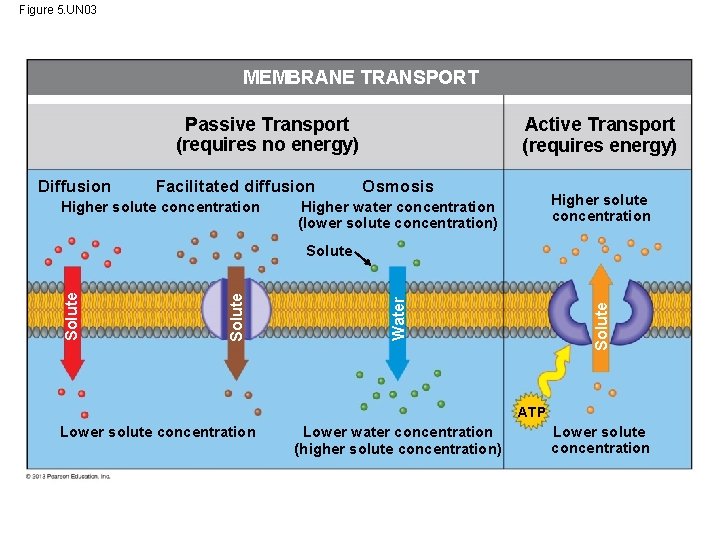
Figure 5. UN 03 MEMBRANE TRANSPORT Passive Transport (requires no energy) Diffusion Facilitated diffusion Higher solute concentration Active Transport (requires energy) Osmosis Higher solute concentration Higher water concentration (lower solute concentration) Solute Water Solute ATP Lower solute concentration Lower water concentration (higher solute concentration) Lower solute concentration
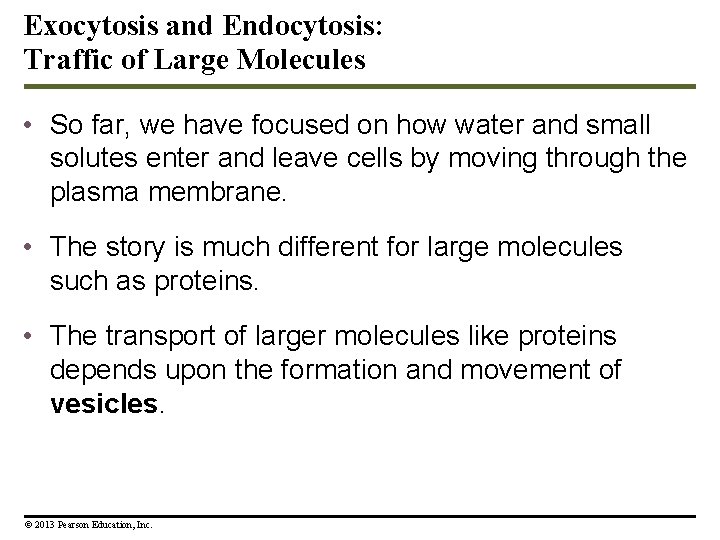
Exocytosis and Endocytosis: Traffic of Large Molecules • So far, we have focused on how water and small solutes enter and leave cells by moving through the plasma membrane. • The story is much different for large molecules such as proteins. • The transport of larger molecules like proteins depends upon the formation and movement of vesicles. © 2013 Pearson Education, Inc.
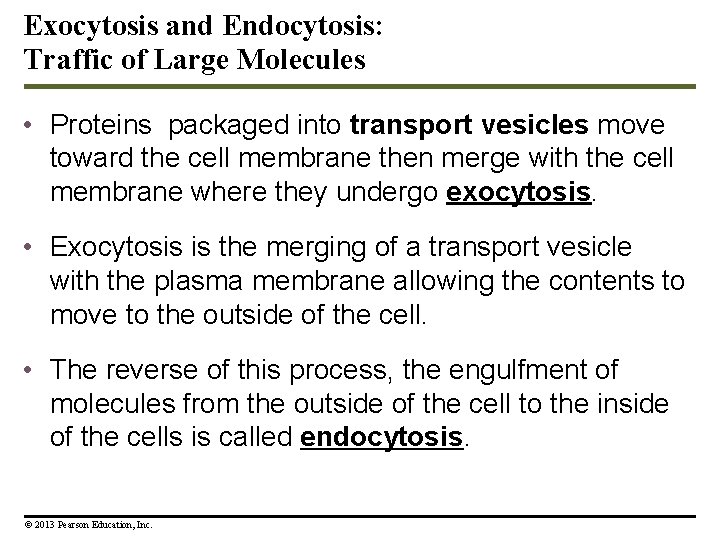
Exocytosis and Endocytosis: Traffic of Large Molecules • Proteins packaged into transport vesicles move toward the cell membrane then merge with the cell membrane where they undergo exocytosis. • Exocytosis is the merging of a transport vesicle with the plasma membrane allowing the contents to move to the outside of the cell. • The reverse of this process, the engulfment of molecules from the outside of the cell to the inside of the cells is called endocytosis. © 2013 Pearson Education, Inc.
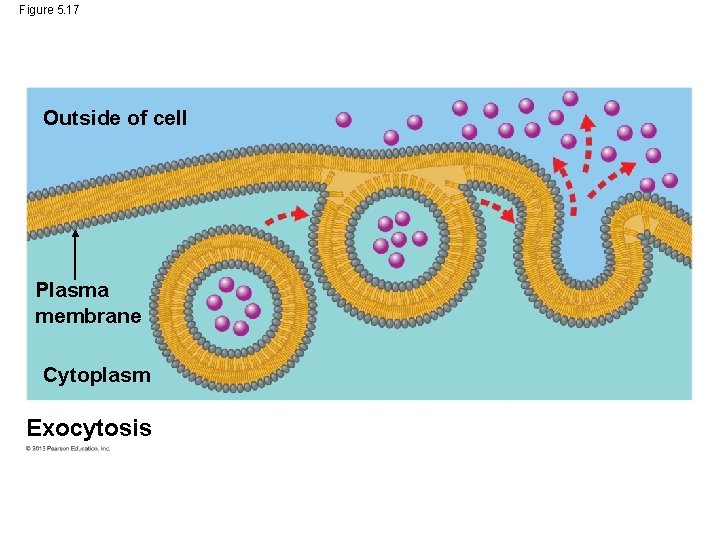
Figure 5. 17 Outside of cell Plasma membrane Cytoplasm Exocytosis
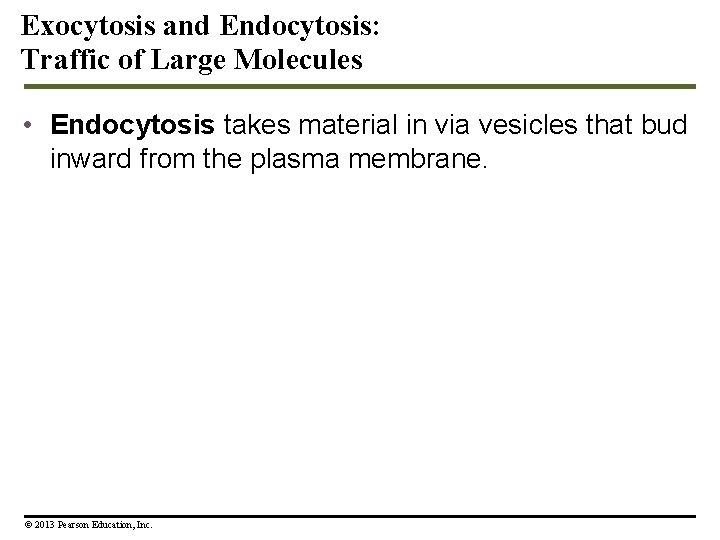
Exocytosis and Endocytosis: Traffic of Large Molecules • Endocytosis takes material in via vesicles that bud inward from the plasma membrane. © 2013 Pearson Education, Inc.
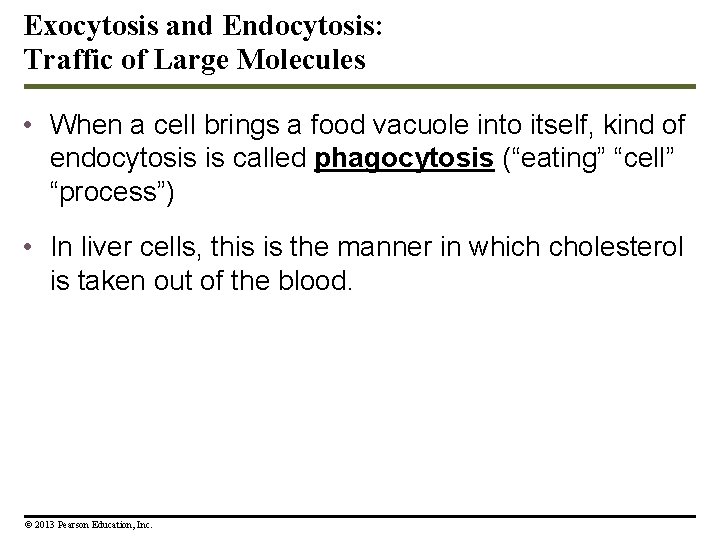
Exocytosis and Endocytosis: Traffic of Large Molecules • When a cell brings a food vacuole into itself, kind of endocytosis is called phagocytosis (“eating” “cell” “process”) • In liver cells, this is the manner in which cholesterol is taken out of the blood. © 2013 Pearson Education, Inc.
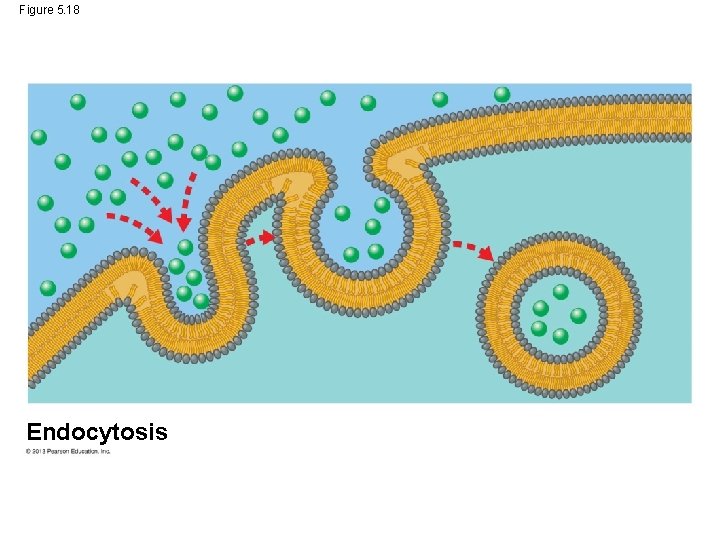
Figure 5. 18 Endocytosis
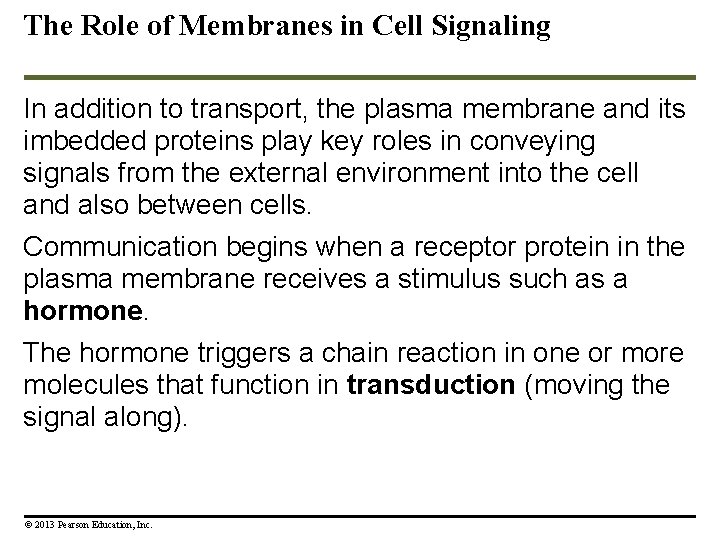
The Role of Membranes in Cell Signaling In addition to transport, the plasma membrane and its imbedded proteins play key roles in conveying signals from the external environment into the cell and also between cells. Communication begins when a receptor protein in the plasma membrane receives a stimulus such as a hormone. The hormone triggers a chain reaction in one or more molecules that function in transduction (moving the signal along). © 2013 Pearson Education, Inc.
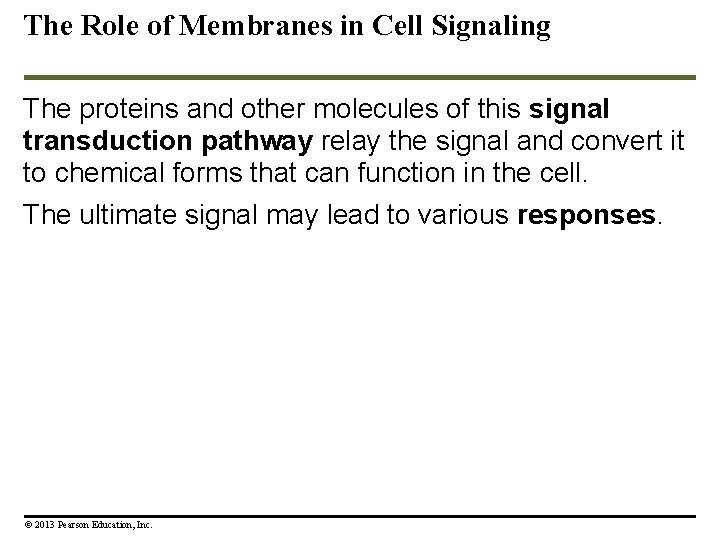
The Role of Membranes in Cell Signaling The proteins and other molecules of this signal transduction pathway relay the signal and convert it to chemical forms that can function in the cell. The ultimate signal may lead to various responses. © 2013 Pearson Education, Inc.
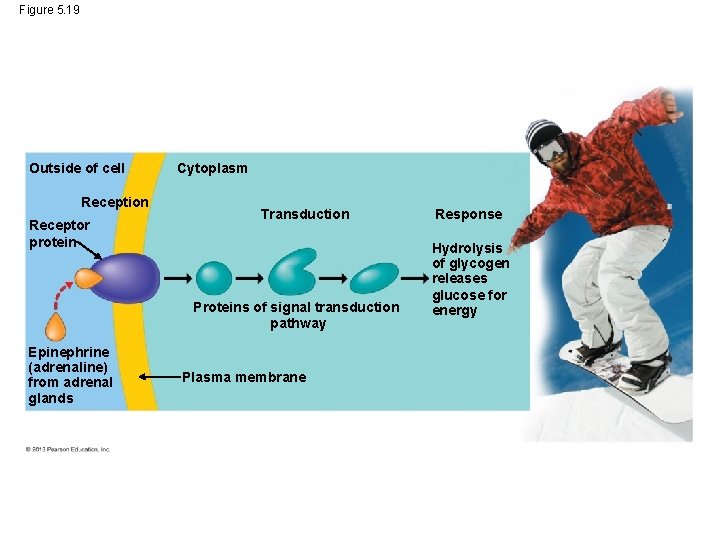
Figure 5. 19 Outside of cell Reception Receptor protein Cytoplasm Transduction Proteins of signal transduction pathway Epinephrine (adrenaline) from adrenal glands Plasma membrane Response Hydrolysis of glycogen releases glucose for energy
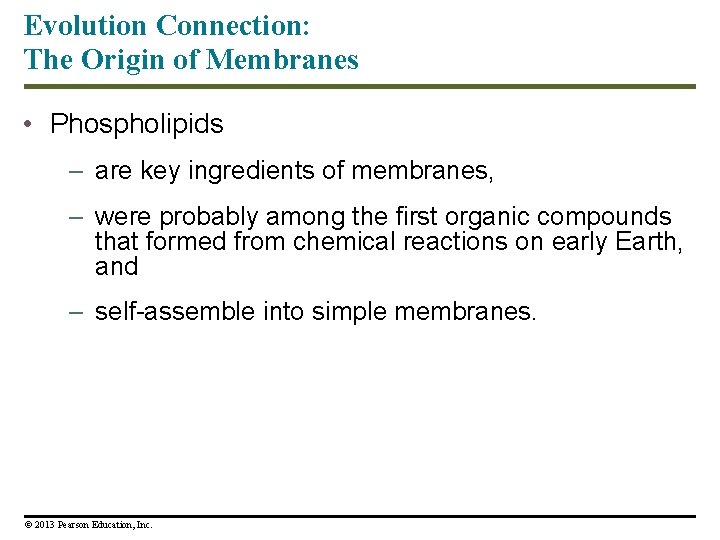
Evolution Connection: The Origin of Membranes • Phospholipids – are key ingredients of membranes, – were probably among the first organic compounds that formed from chemical reactions on early Earth, and – self-assemble into simple membranes. © 2013 Pearson Education, Inc.
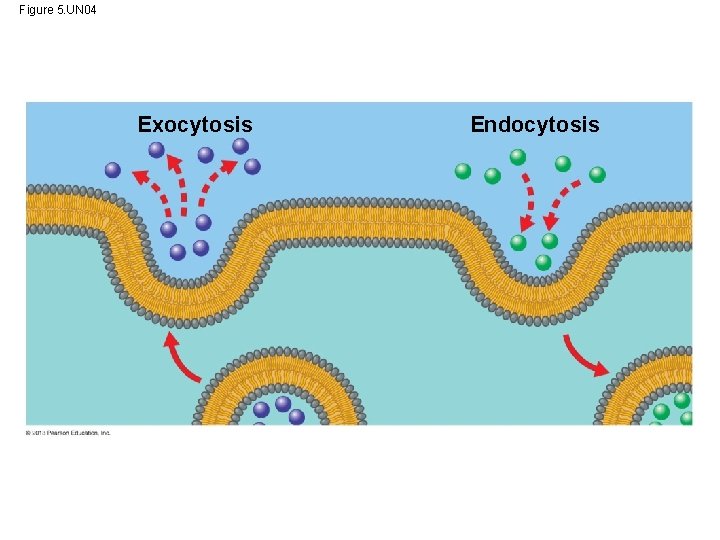
Figure 5. UN 04 Exocytosis Endocytosis
Molecular biology lectures
Power system lectures
Chapter 5 the working cell
Power triangle formula
Powerbi in powerpoint
Hard work and smart work
Hot metal forming
Hot working and cold working difference
Differentiate between hot working and cold working
Pembentukan plat pada pengerjaan panas
Point point power
The scientist mathias schleiden studied _______ in ______.
Rick trebino lectures
Lectures paediatrics
Data mining lectures
Advanced medicinal chemistry
Uva powerpoint template
Ludic space
Activity identification approaches in spm
Radio astronomy lectures
Dr sohail lectures
Utilities and energy lectures
Web engineering lectures ppt
How to get the most out of lectures
Frcr physics lectures
Frequency of xrays
Introduction to recursion
Rbc formation stages
What is aerodynamics
Tamara berg husband
What is text linguistics
Theory of translation lectures
Digital logic design lectures
Kurose ross computer networking
Hegel three forms of art
Nuclear medicine lectures
Gpoint c++
Cdeep lectures
Oral communication 3 lectures text
C programming and numerical analysis an introduction
Haematology lectures
Bureau of lectures
Slagle lecture
Theory of translation lectures
Reinforcement learning lectures
13 lectures
Reinforcement learning lectures
Bba lectures
Medical emergency student lectures
Medical hematology student lectures
Rcog eportfolio
Bhadeshia lectures
Ota core curriculum
Comsats virtual campus lectures
Hugh blair lectures on rhetoric
Cern summer student lectures
Pathology lectures for medical students
Dr asim lectures
Ota resident lectures
Anatomy lectures powerpoint
Cern summer school lectures
References
Power query intellisense not working
Diesel engine power plant working animation
Power curve working group
Power curve working group
Hát kết hợp bộ gõ cơ thể
Slidetodoc
Bổ thể
Tỉ lệ cơ thể trẻ em
Voi kéo gỗ như thế nào
Tư thế worms-breton
Chúa sống lại
Các môn thể thao bắt đầu bằng tiếng nhảy
Thế nào là hệ số cao nhất
Các châu lục và đại dương trên thế giới
Công thức tính độ biến thiên đông lượng
Trời xanh đây là của chúng ta thể thơ
Cách giải mật thư tọa độ
Phép trừ bù
Phản ứng thế ankan
Các châu lục và đại dương trên thế giới
Thể thơ truyền thống
Quá trình desamine hóa có thể tạo ra
Một số thể thơ truyền thống
Cái miệng xinh xinh thế chỉ nói điều hay thôi
Vẽ hình chiếu vuông góc của vật thể sau
Nguyên nhân của sự mỏi cơ sinh 8
đặc điểm cơ thể của người tối cổ
Thế nào là giọng cùng tên? *
Vẽ hình chiếu đứng bằng cạnh của vật thể
Phối cảnh
Thẻ vin
đại từ thay thế
điện thế nghỉ
Tư thế ngồi viết
Diễn thế sinh thái là
Dot
So nguyen to
Tư thế ngồi viết
Lời thề hippocrates