The Structure of Atom Thomsons Atomic Model 1897
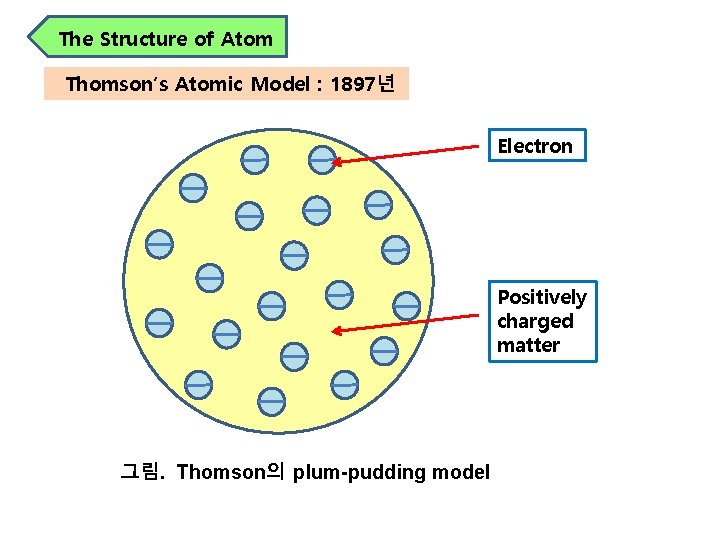
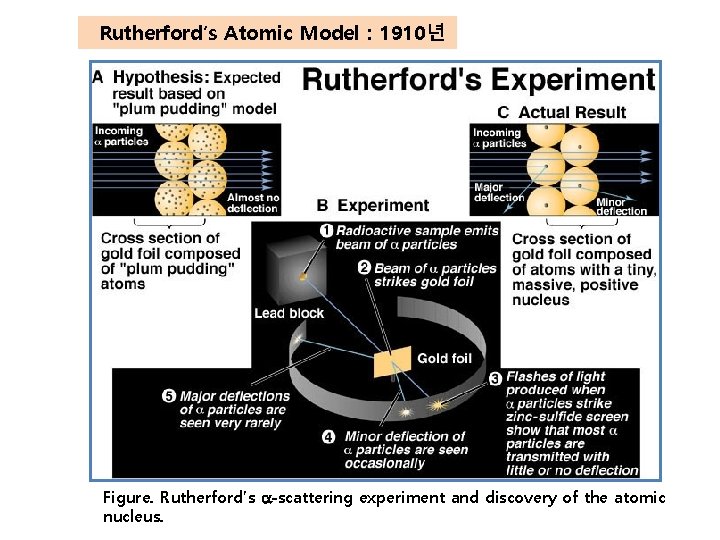
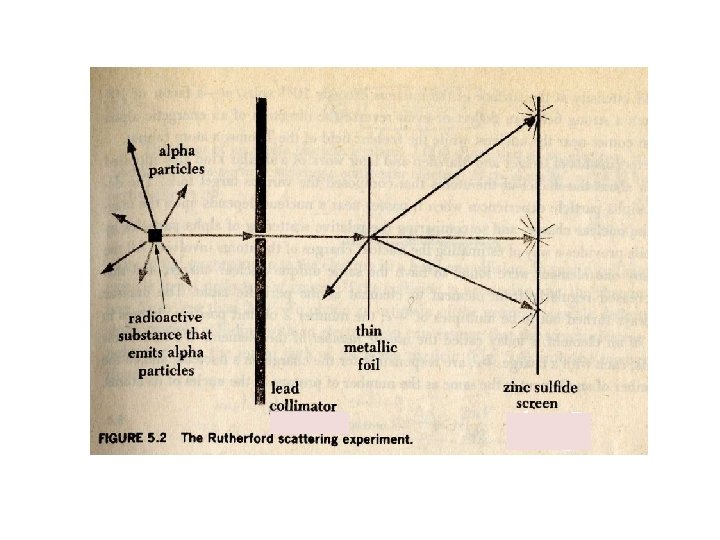
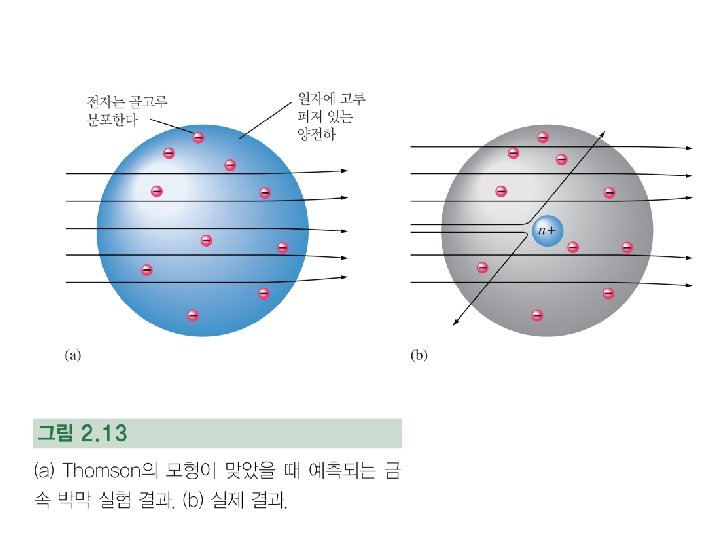
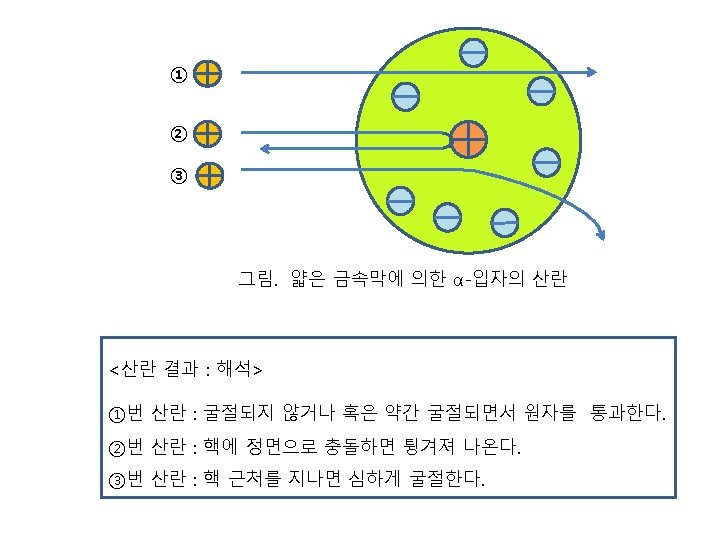
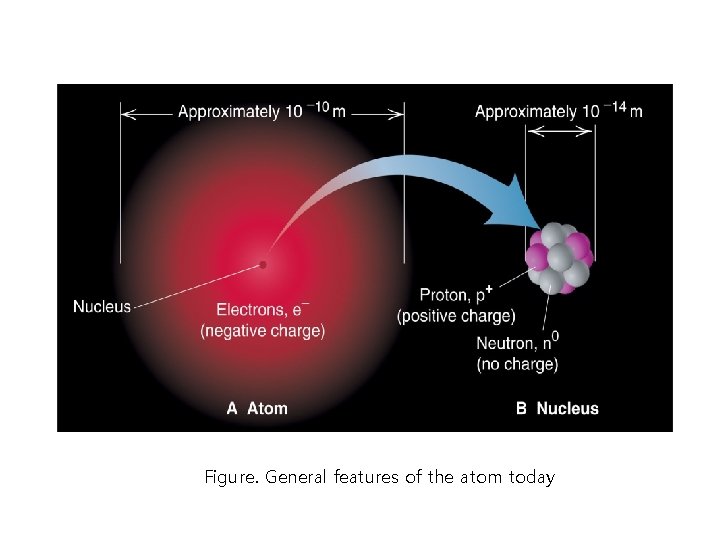
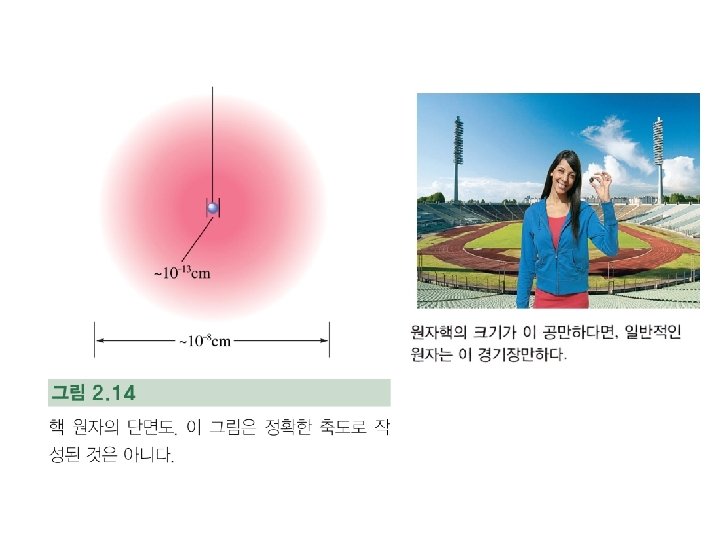
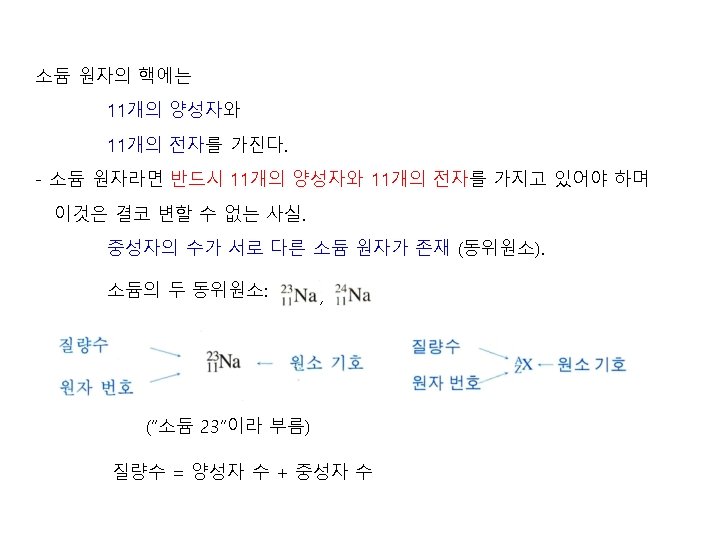
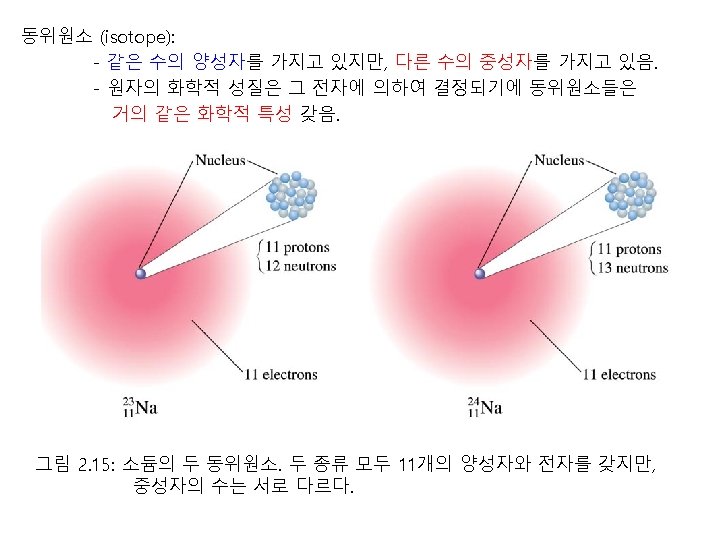
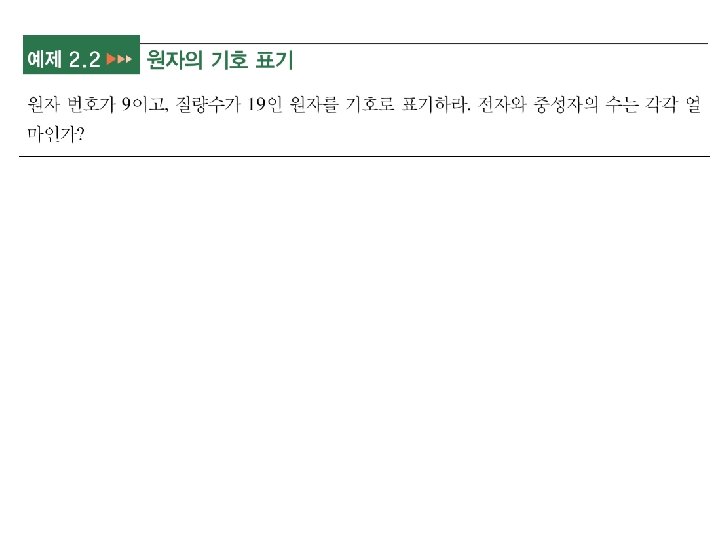
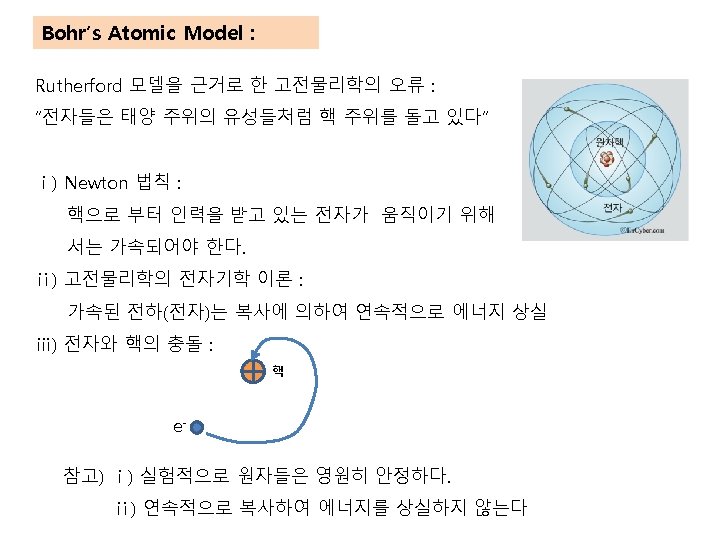
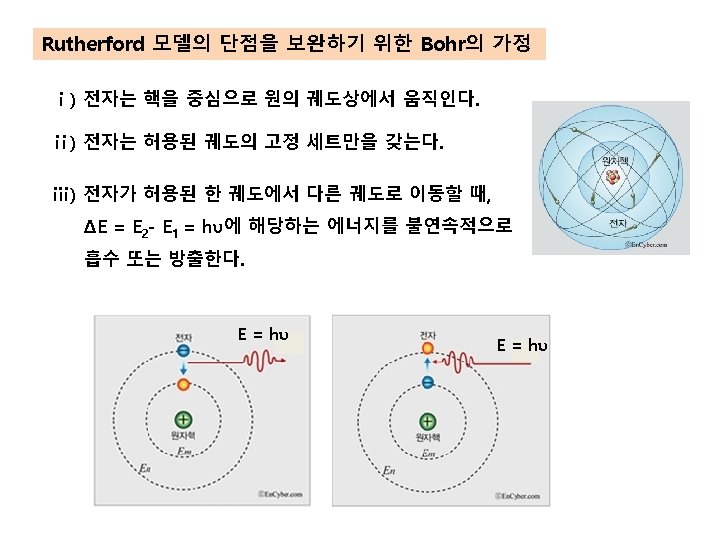
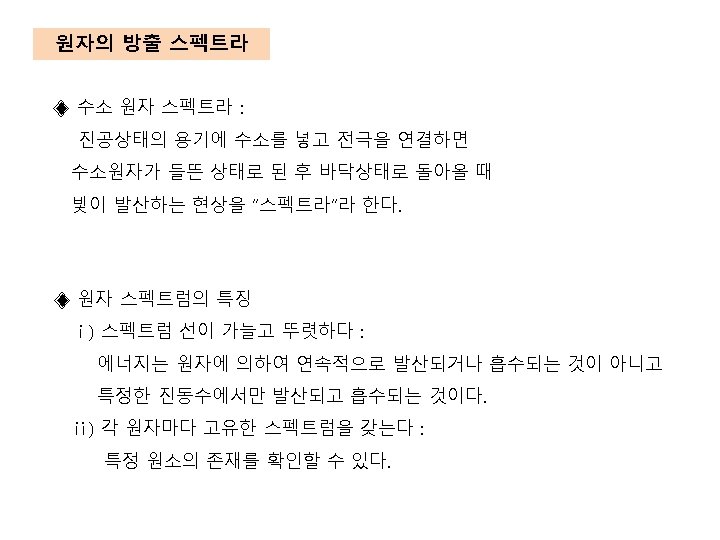
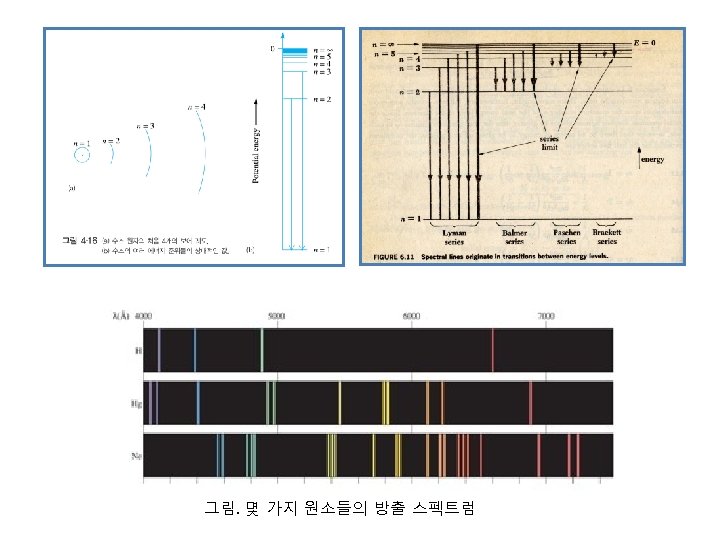
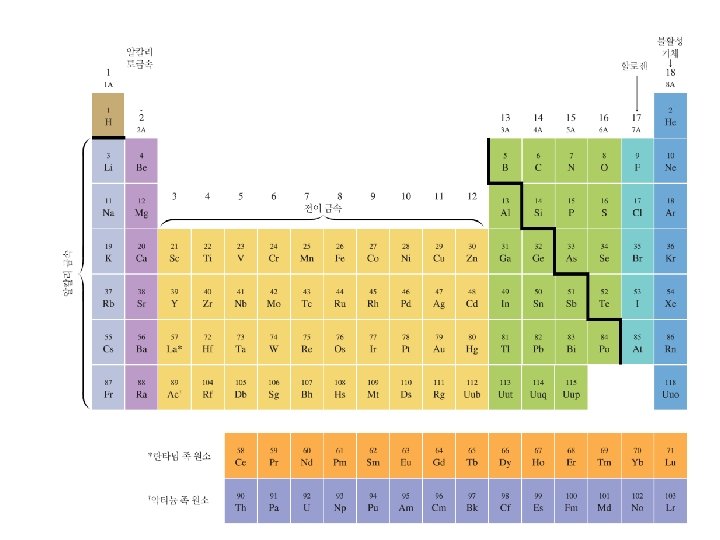
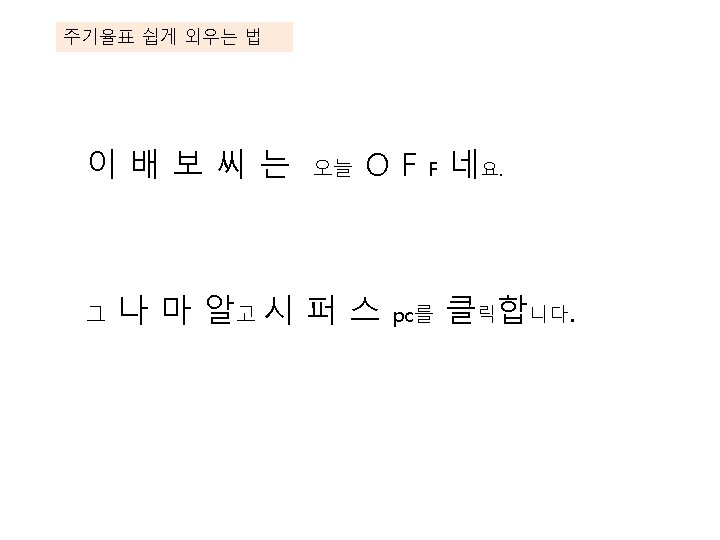
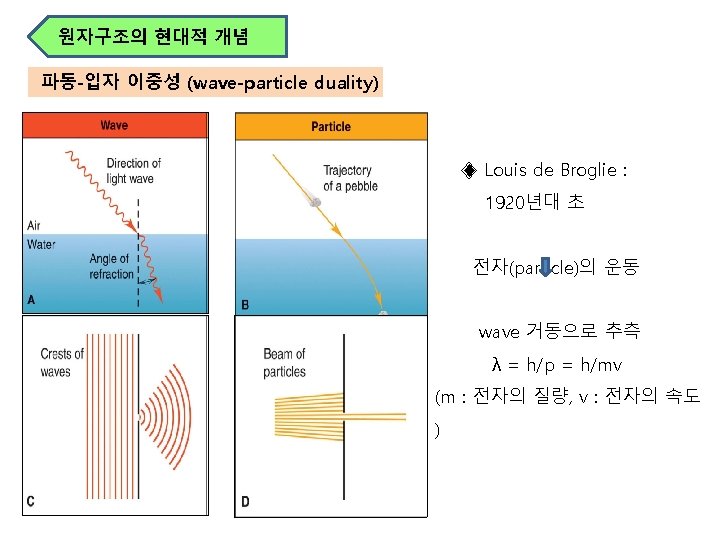
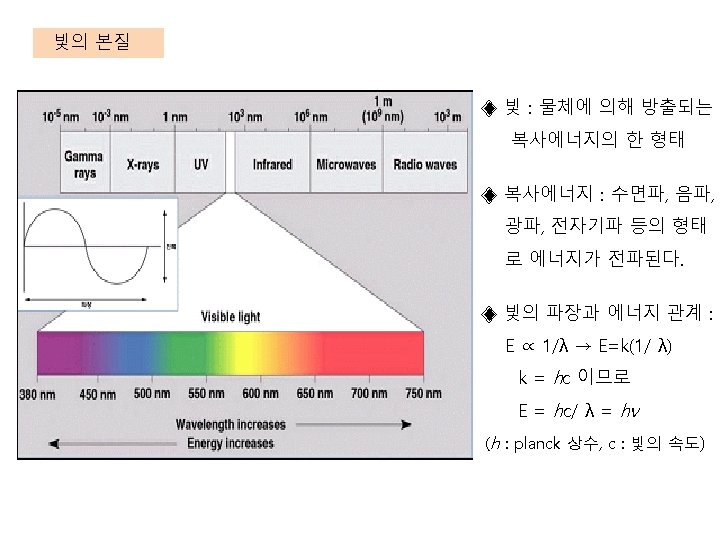
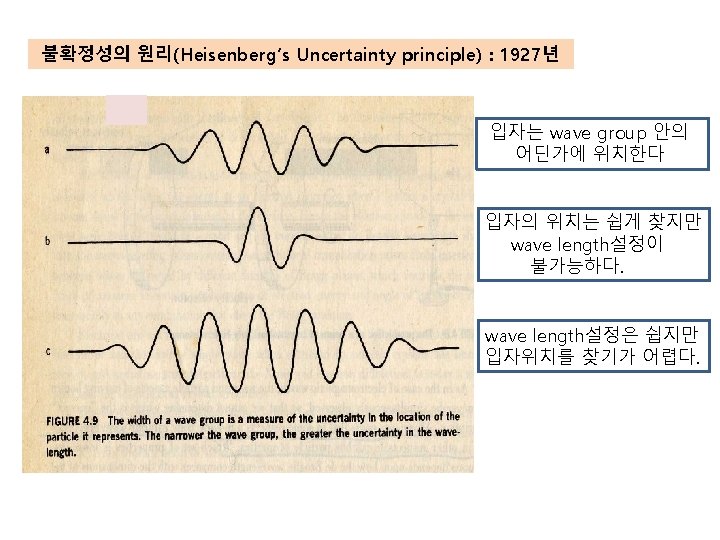
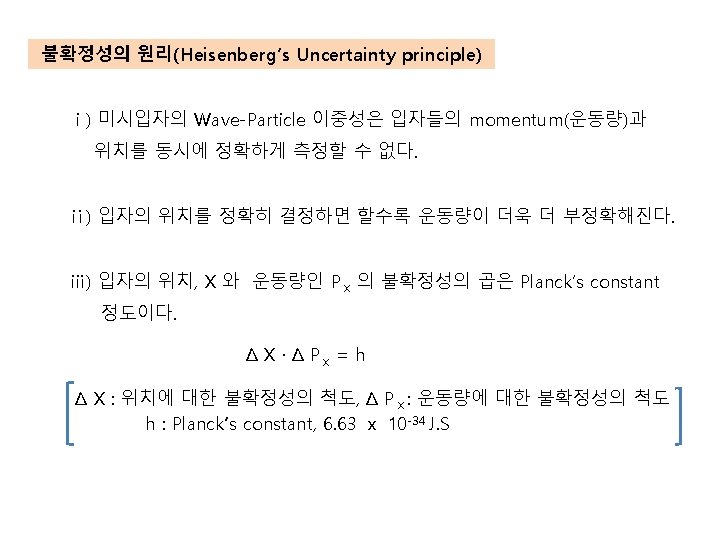
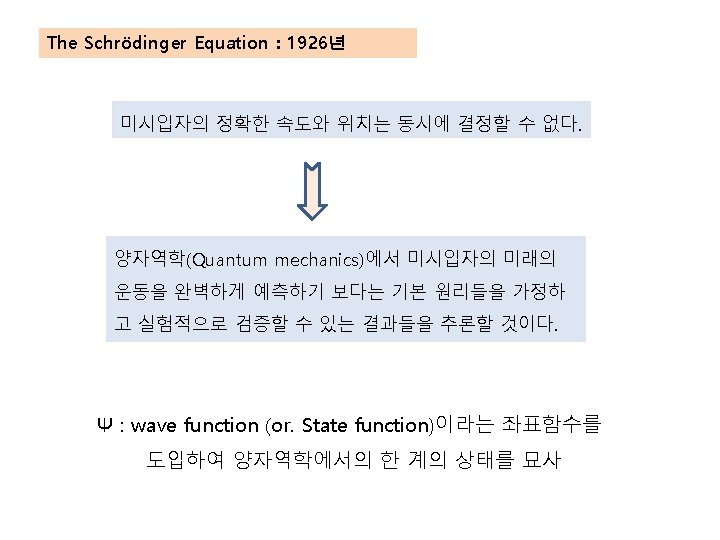
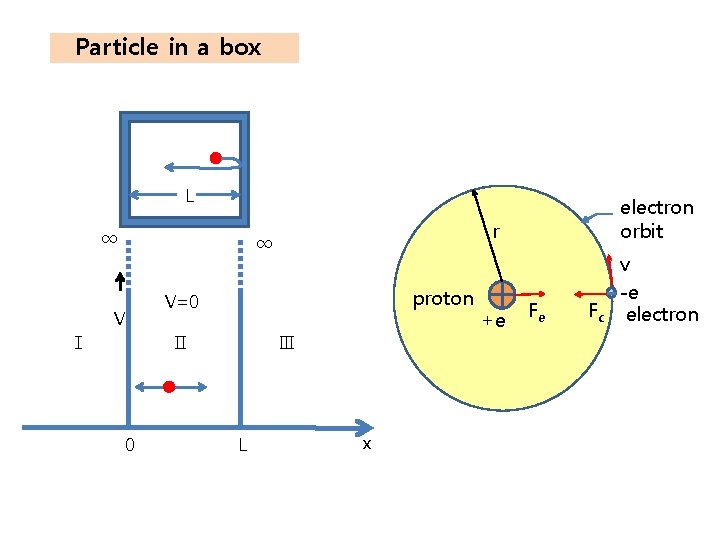
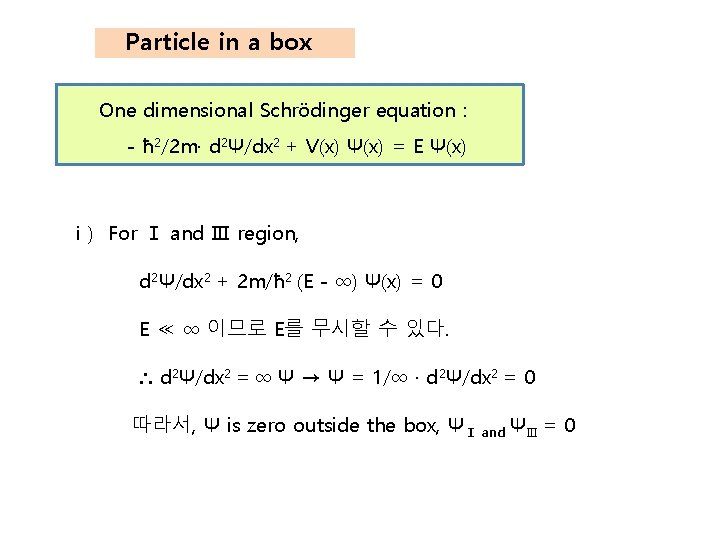
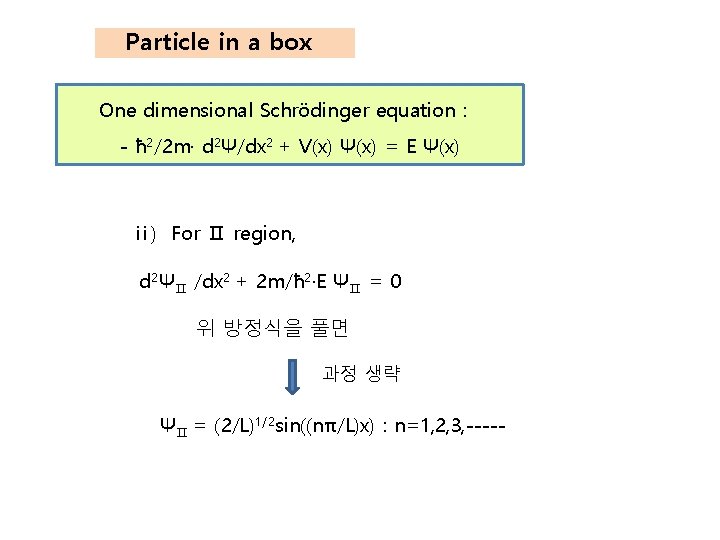
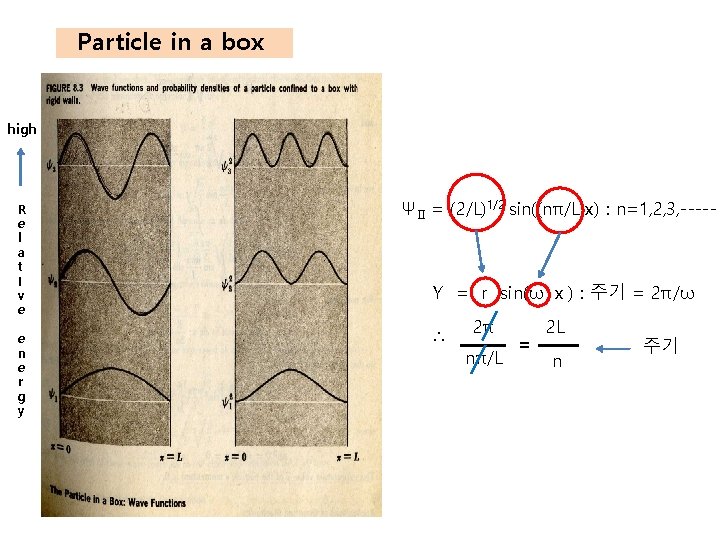
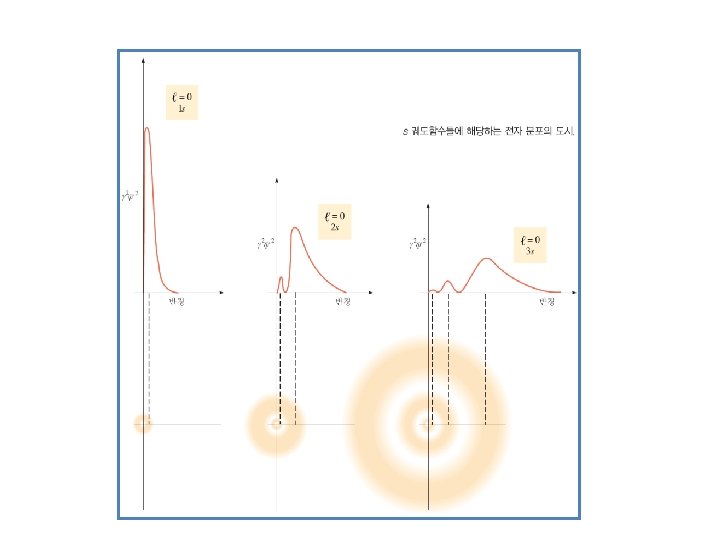
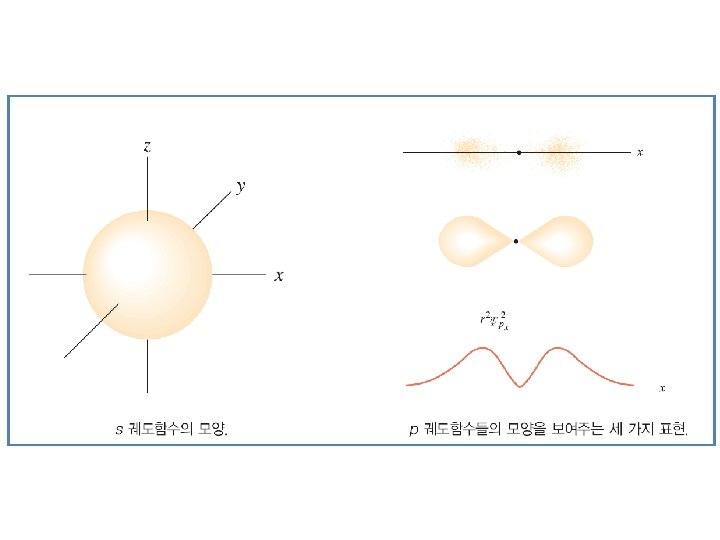
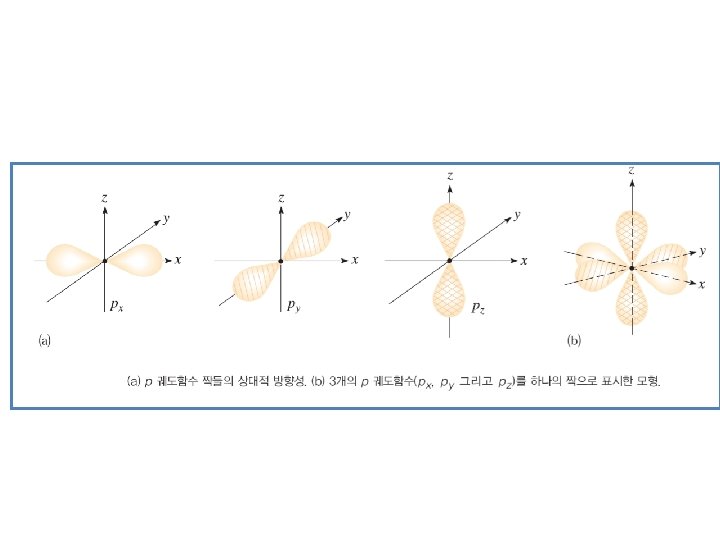
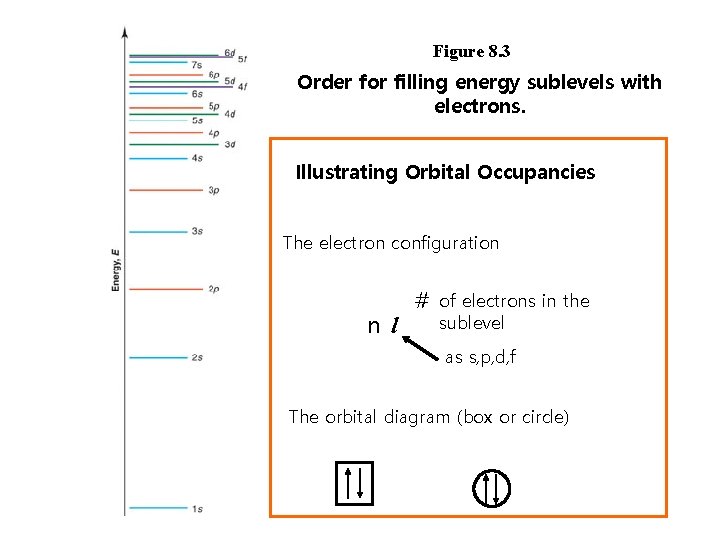
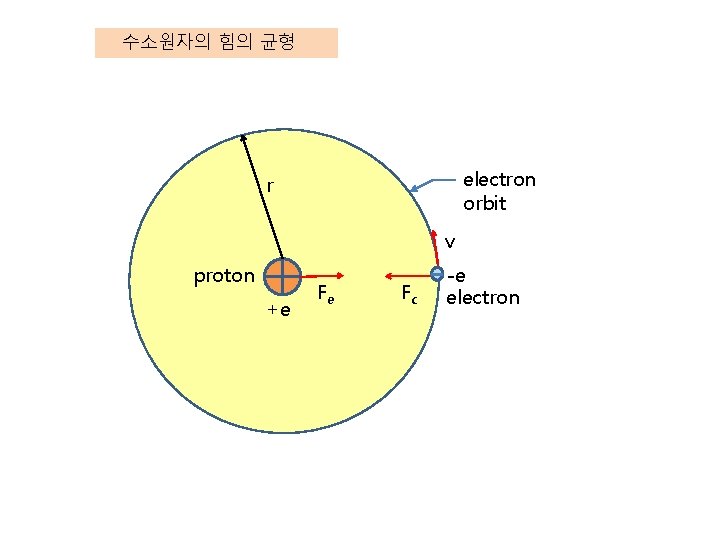
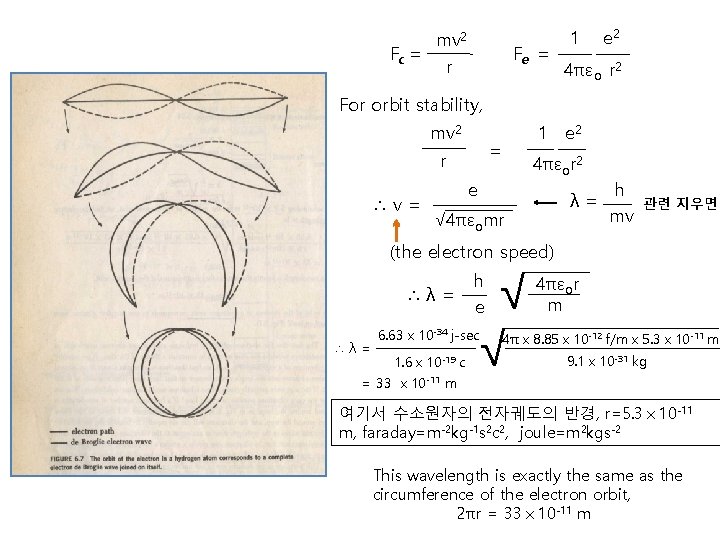
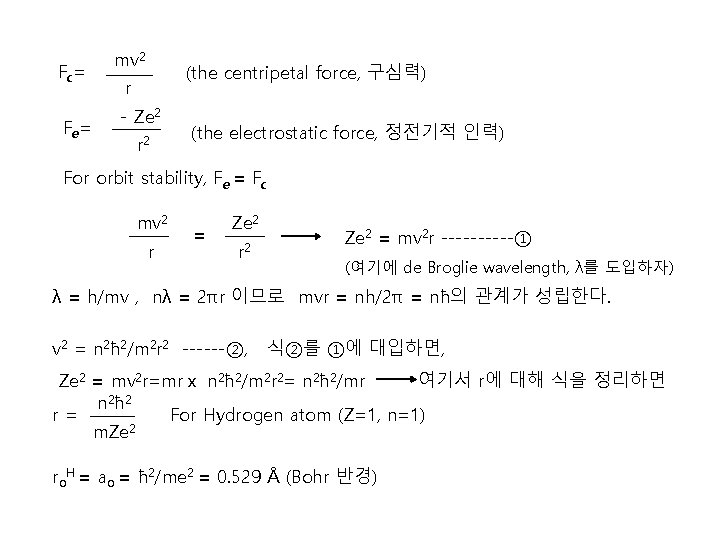
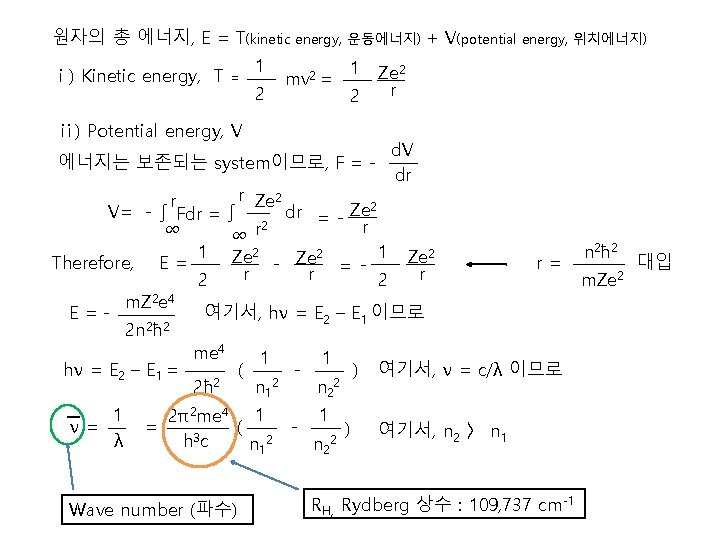
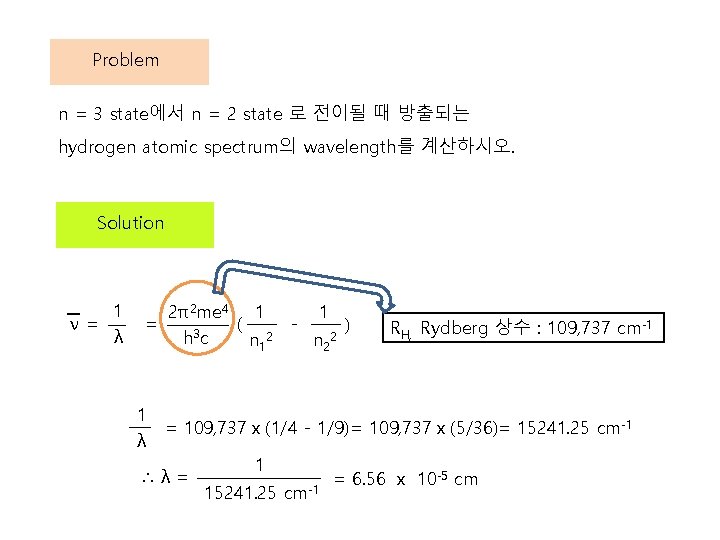
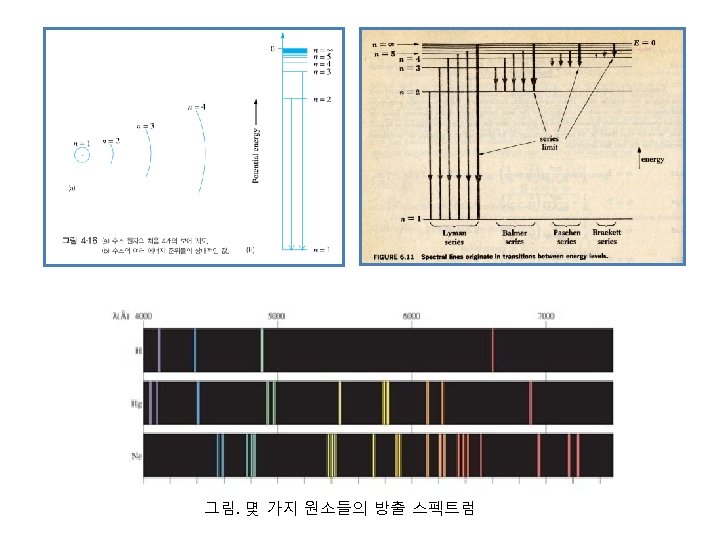
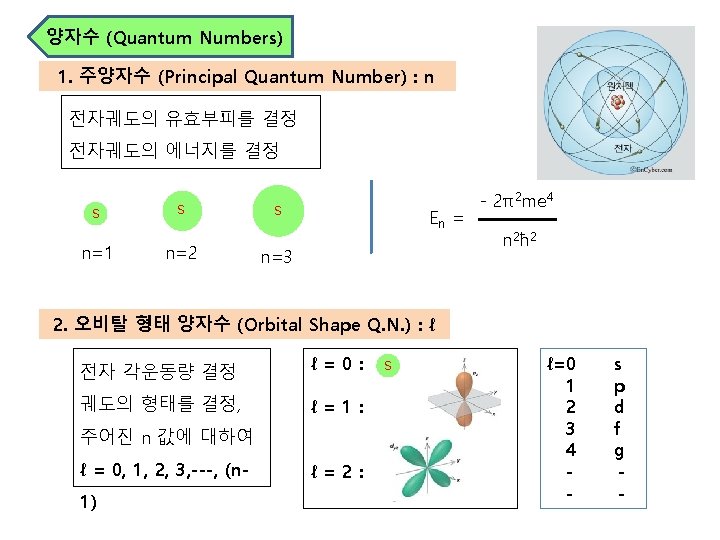
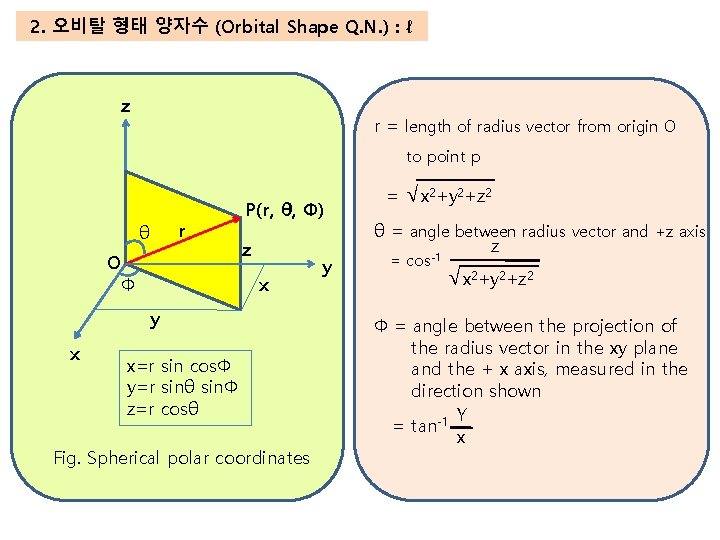
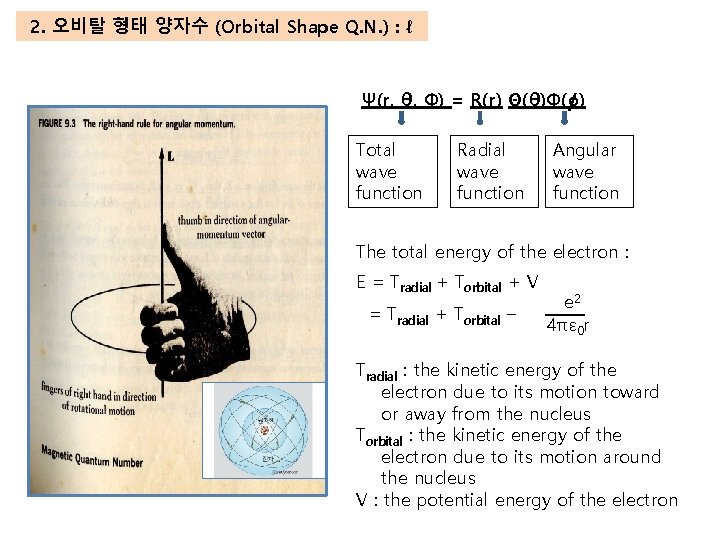
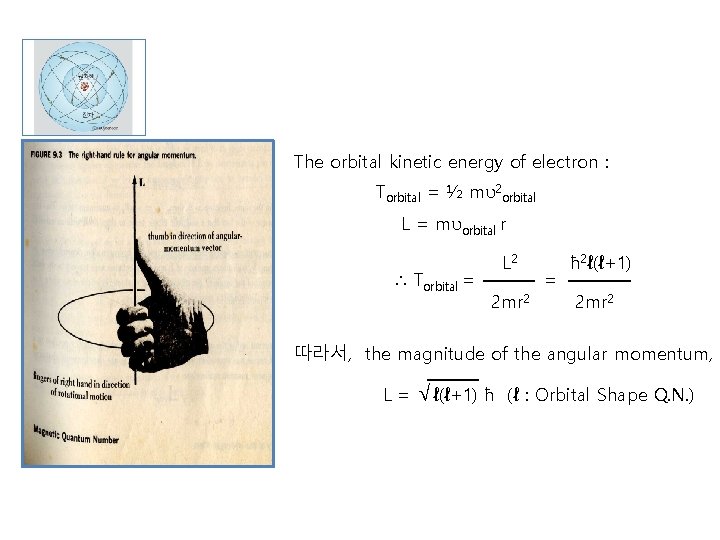
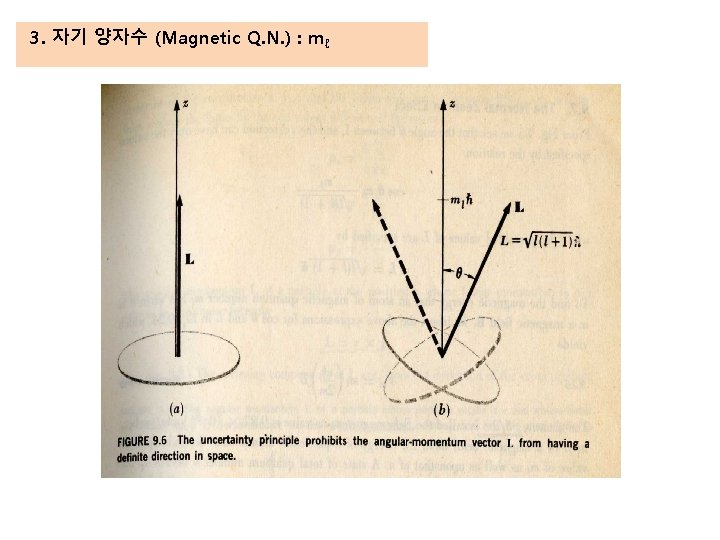
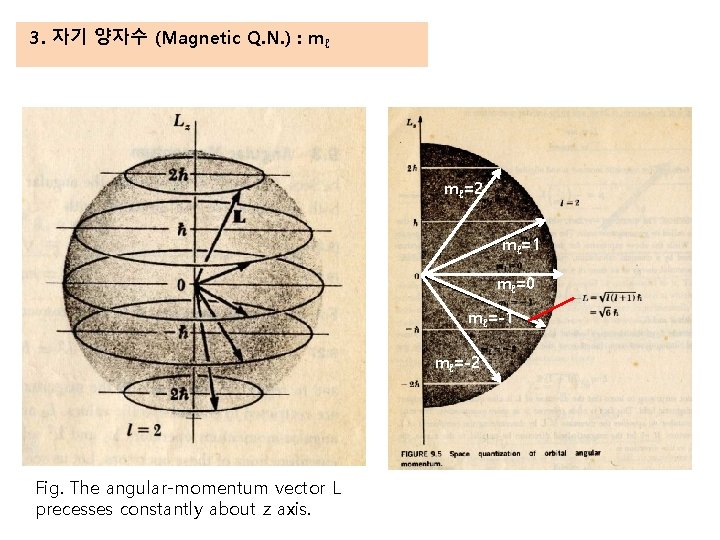
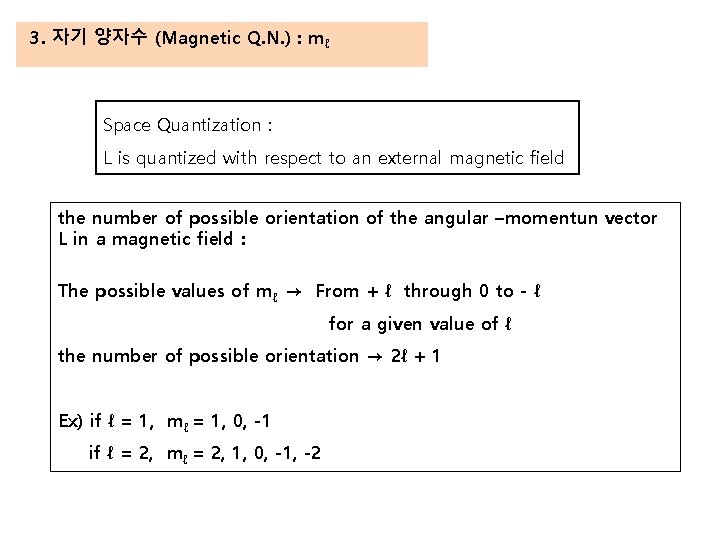
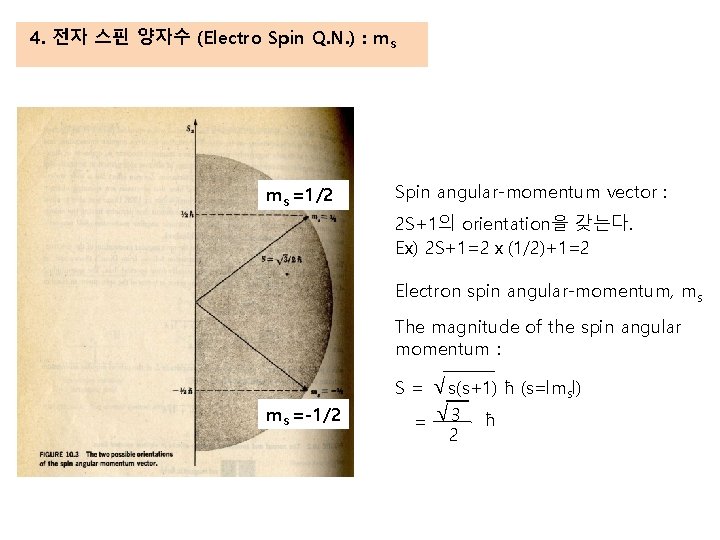
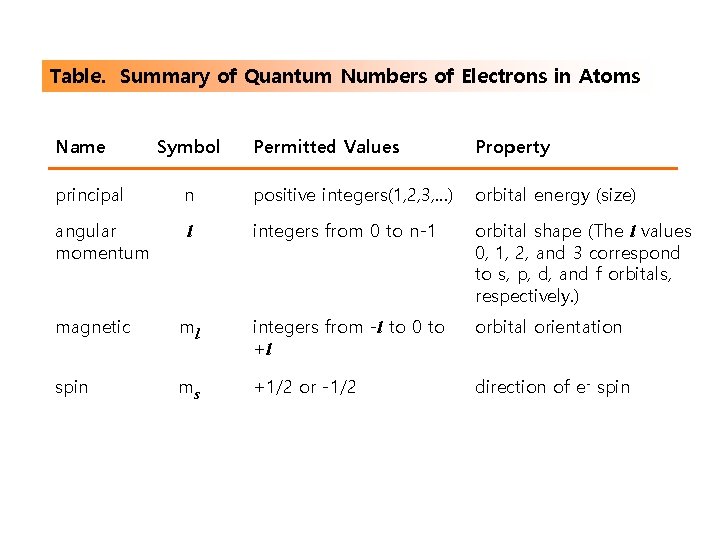
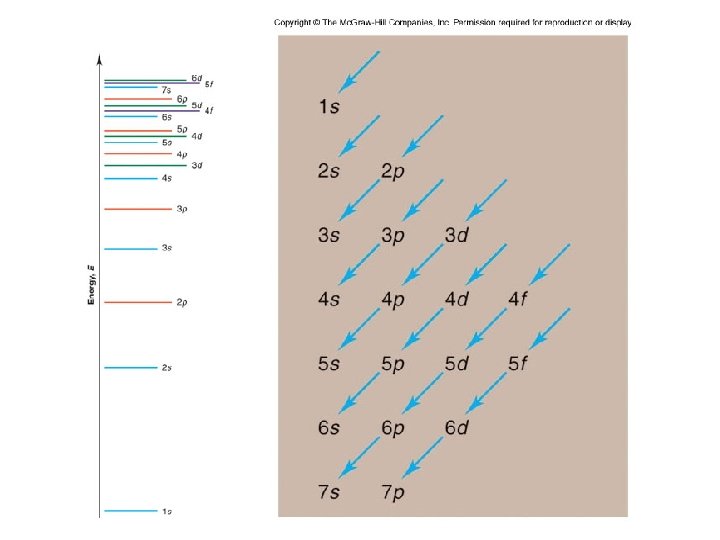
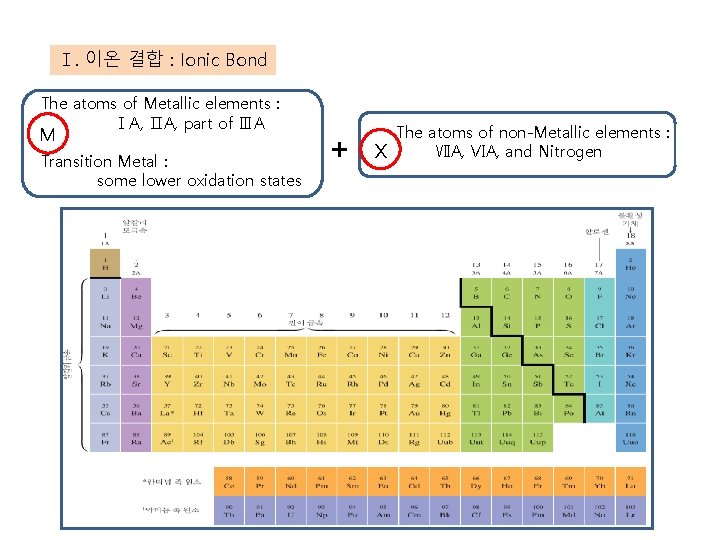
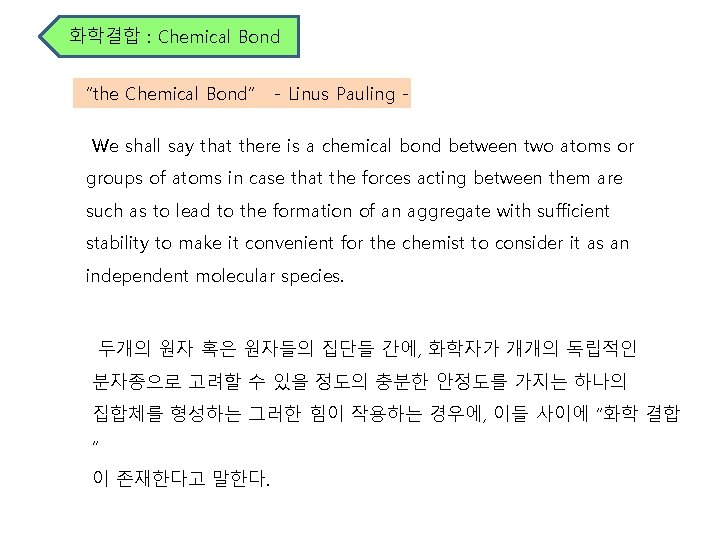
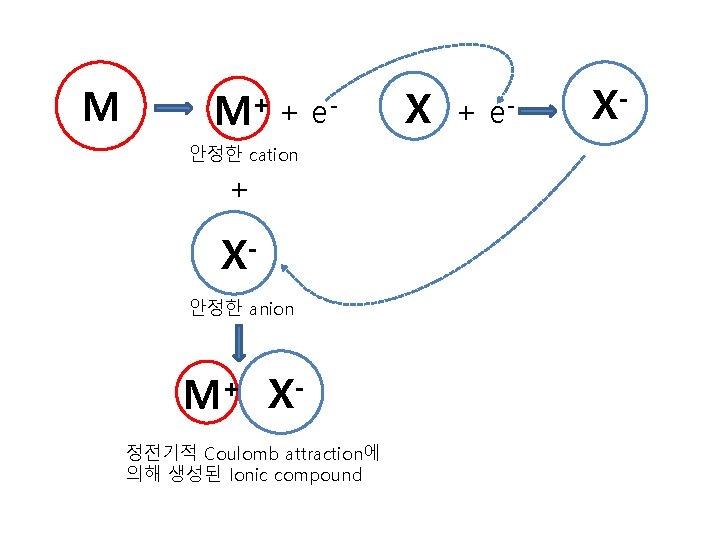
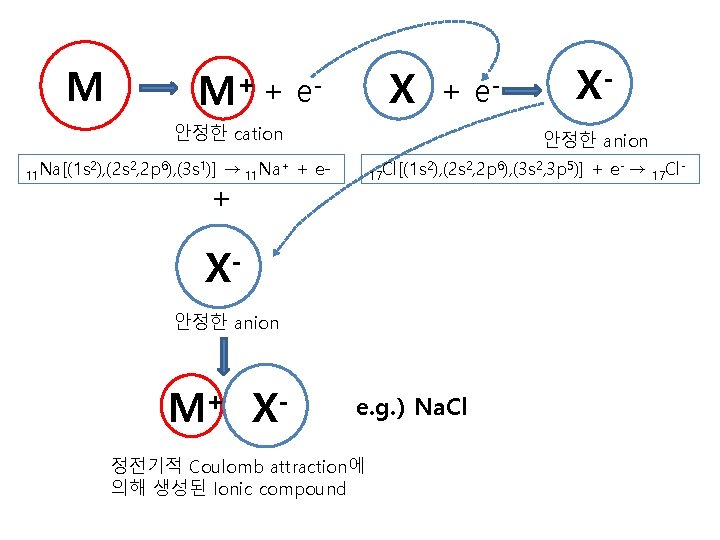
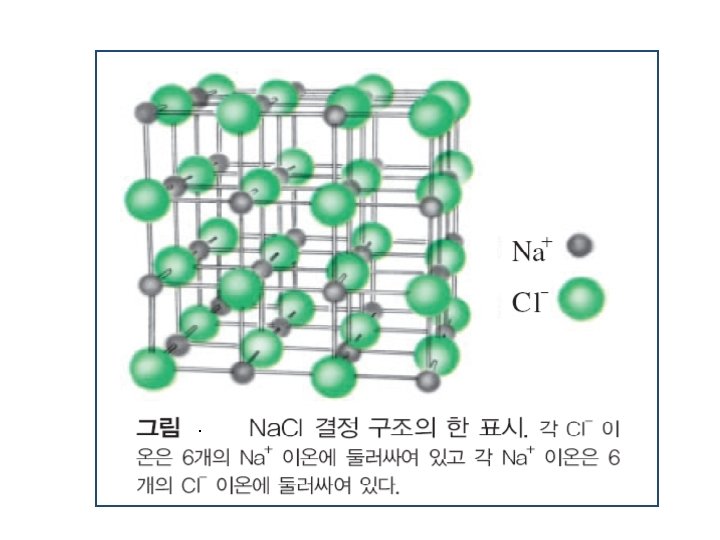
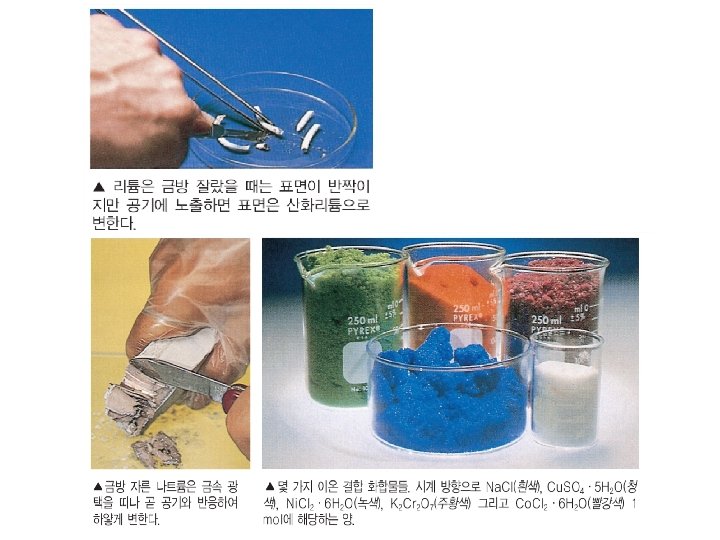
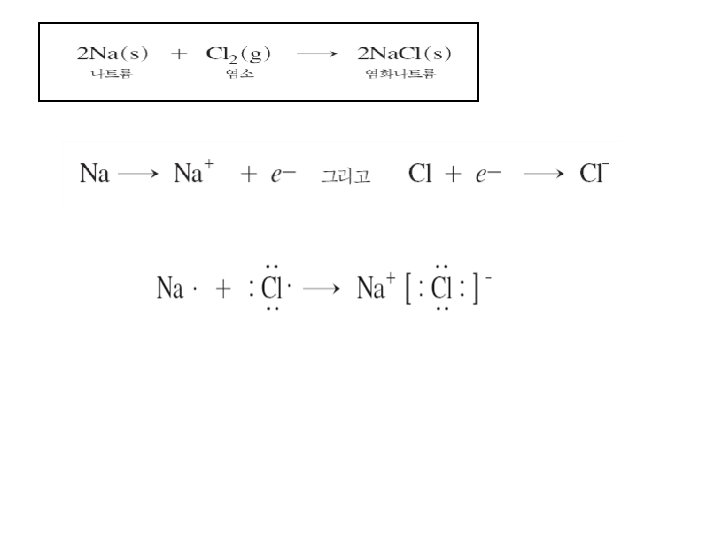
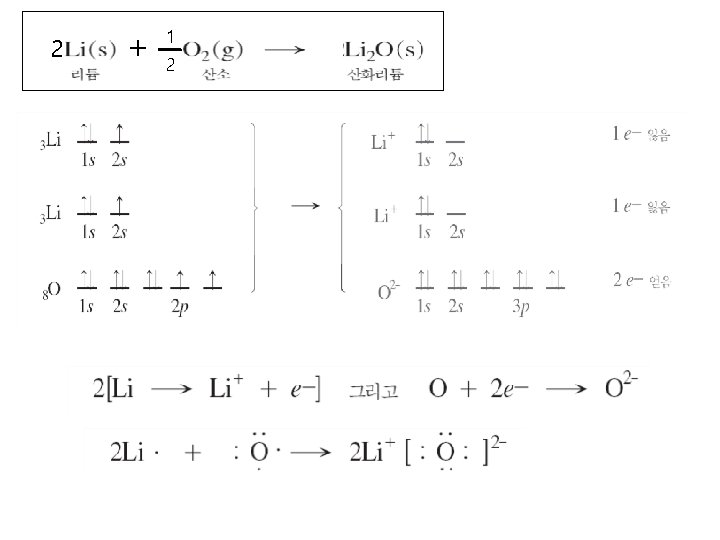
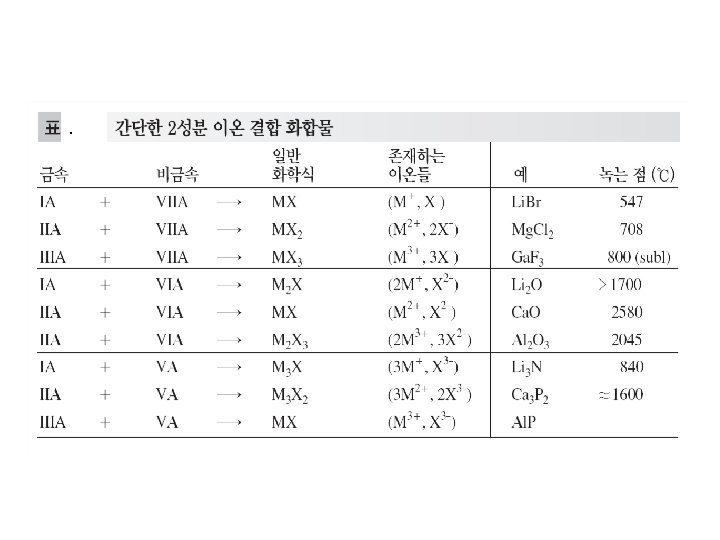
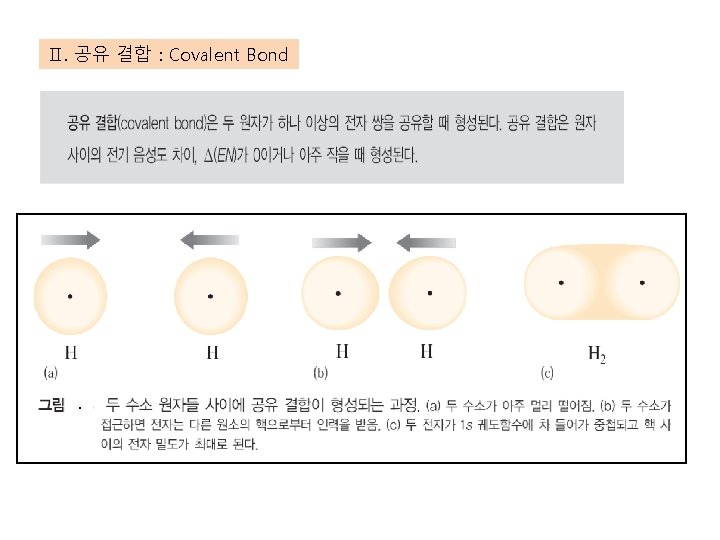
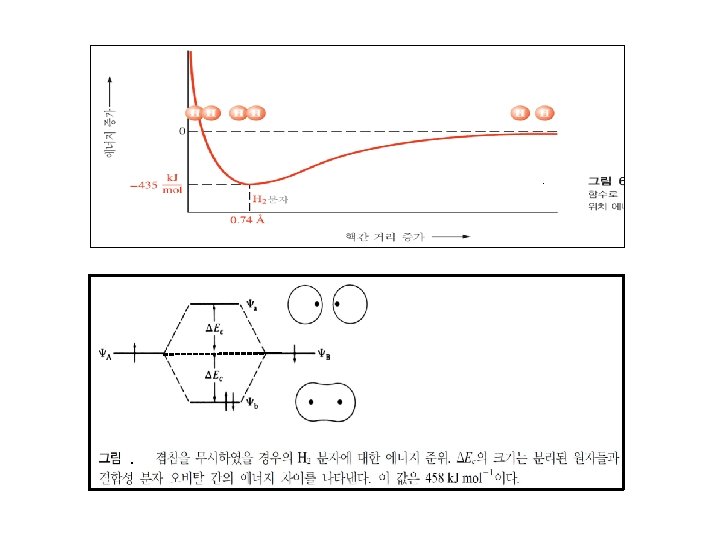
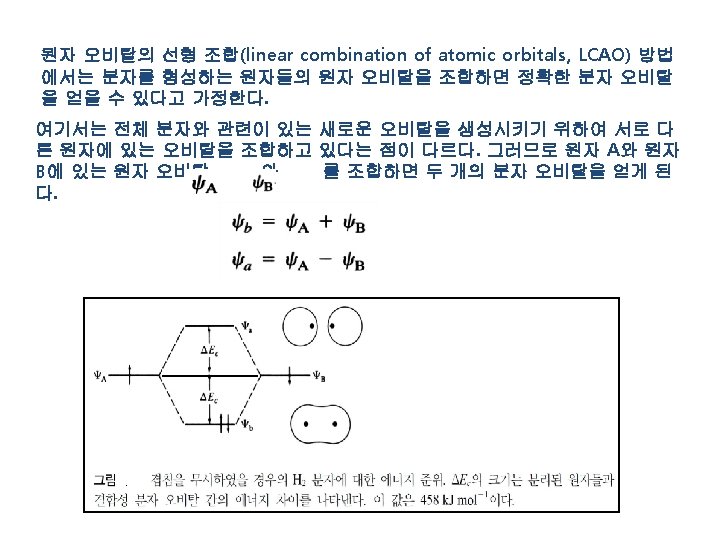
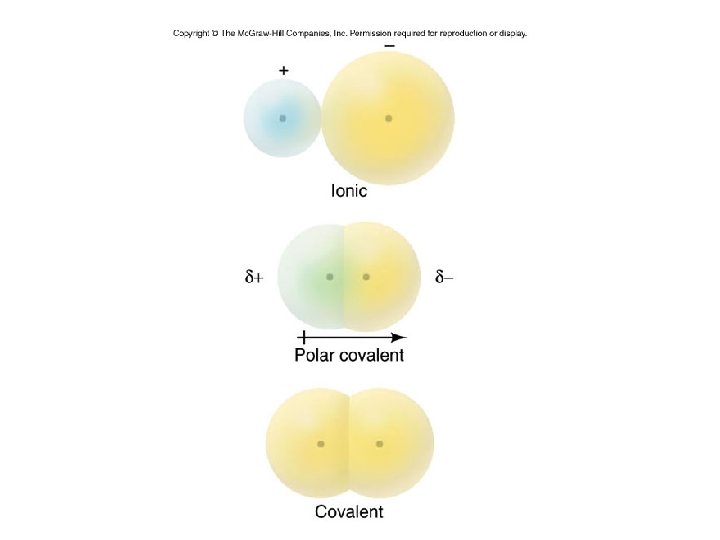
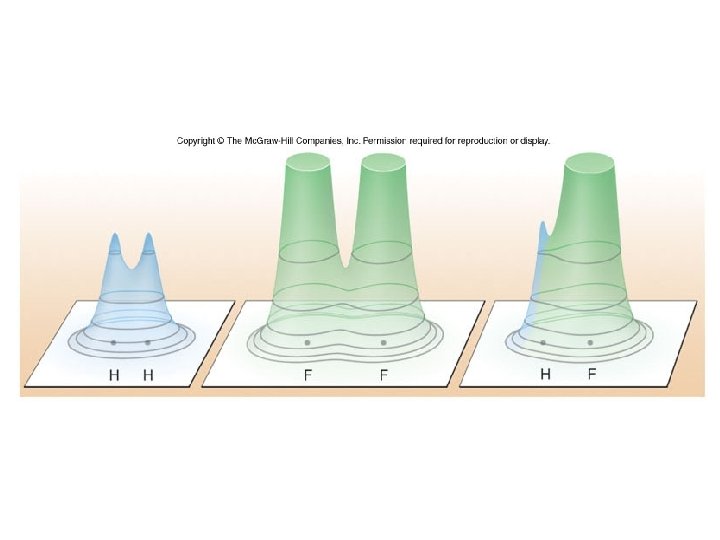
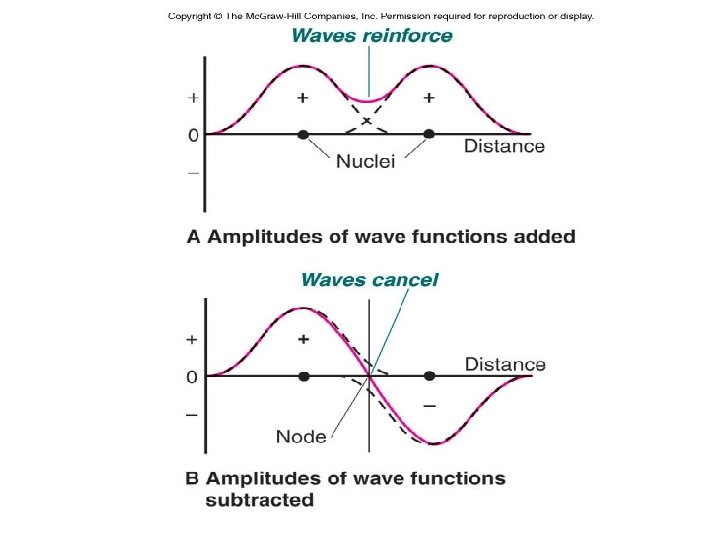
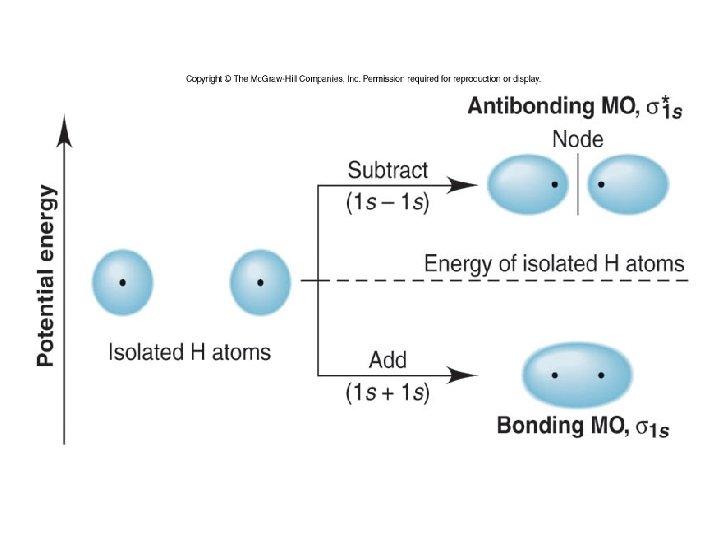
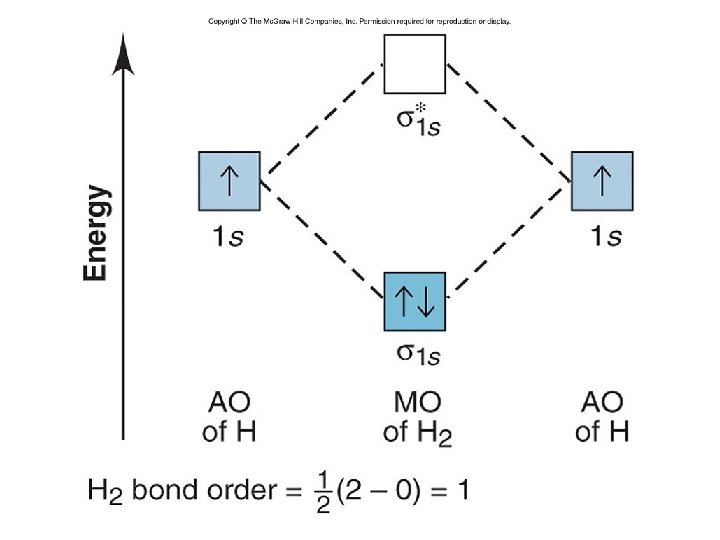
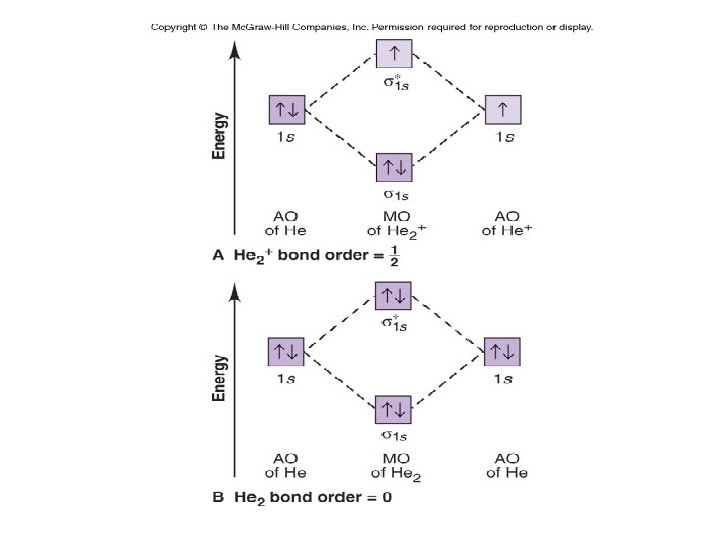
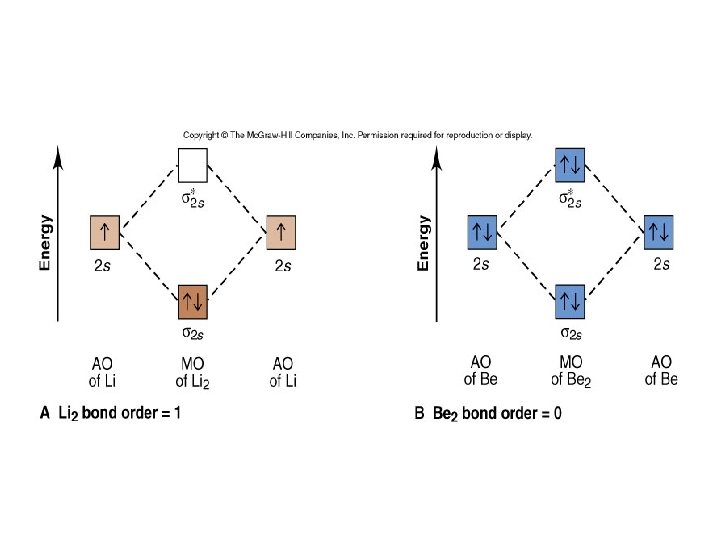
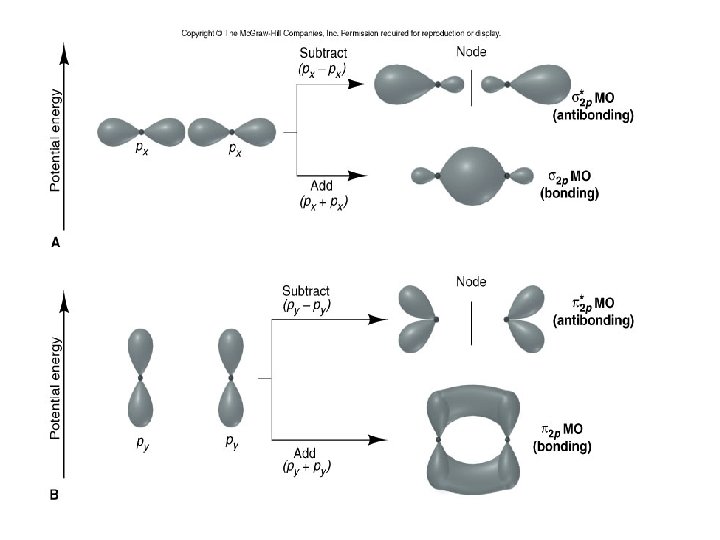
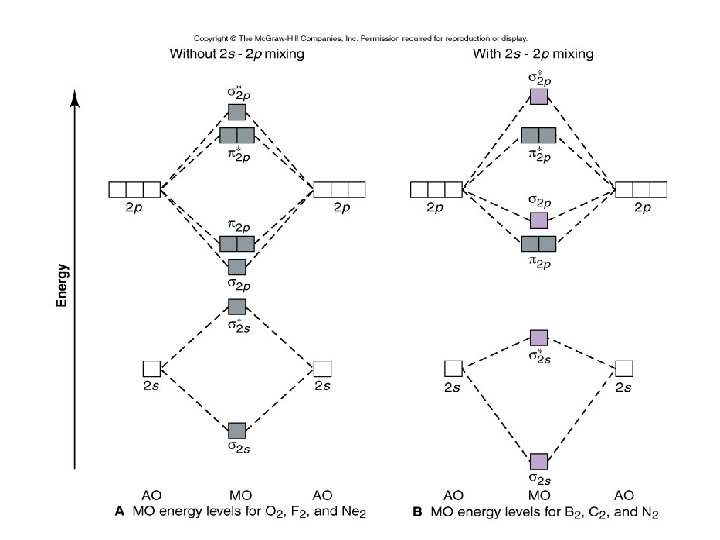
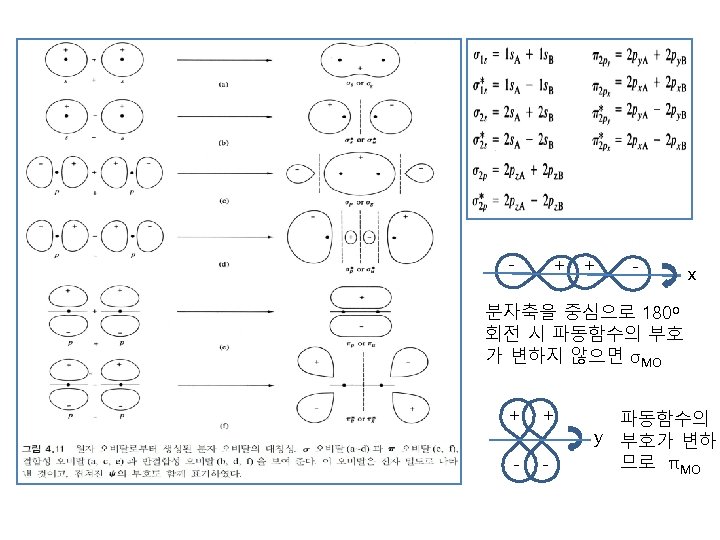
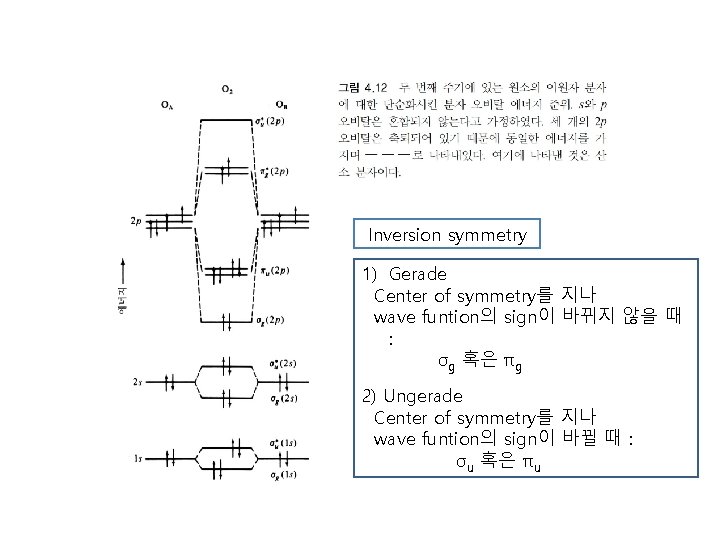
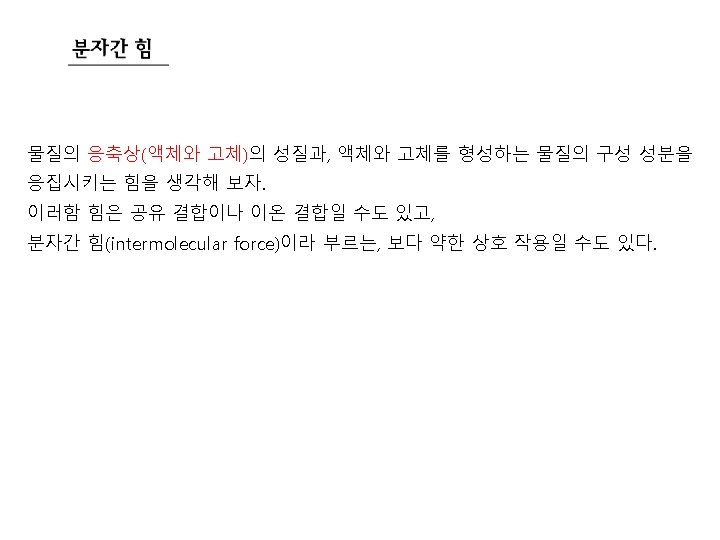
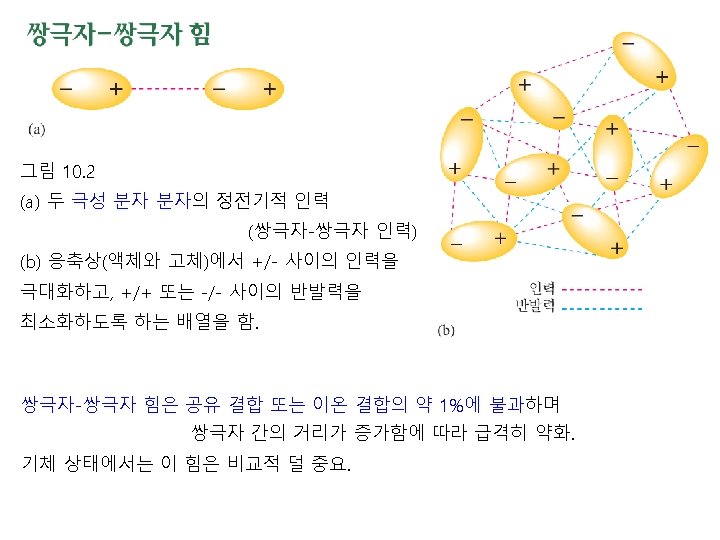
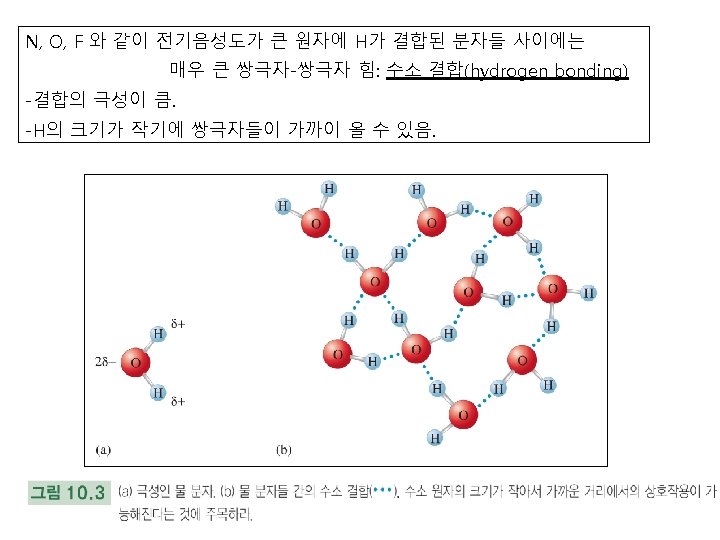
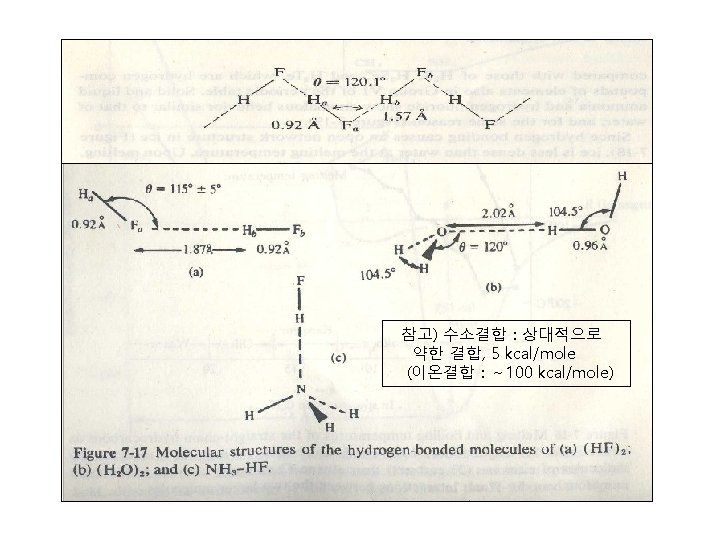
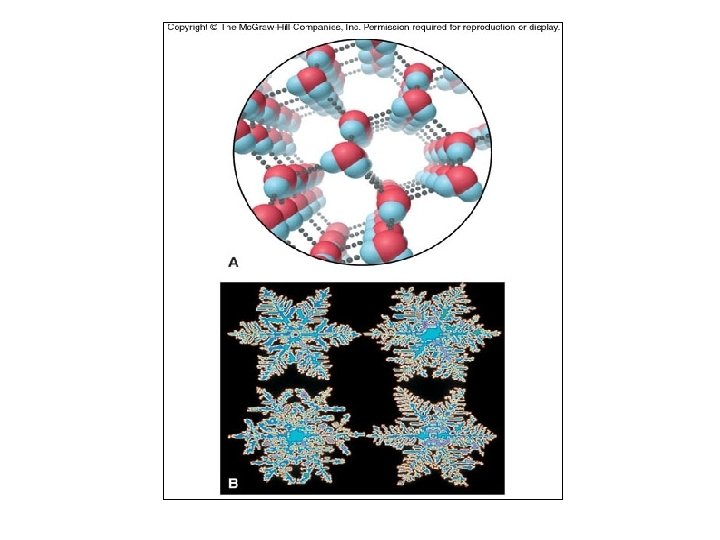
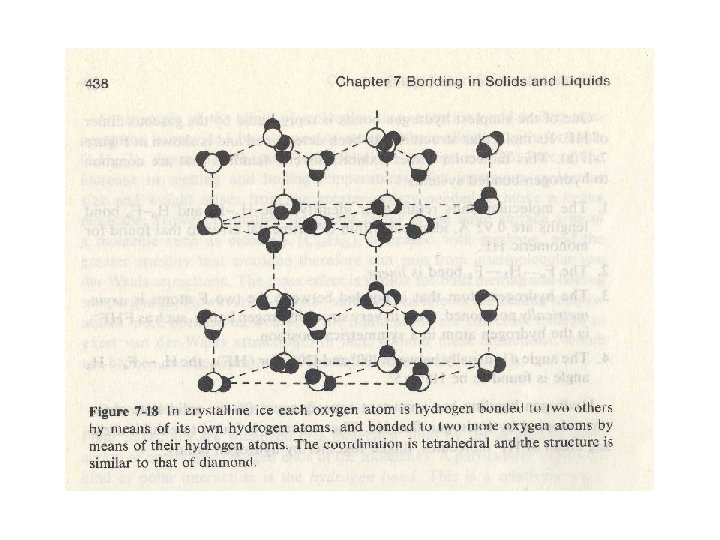
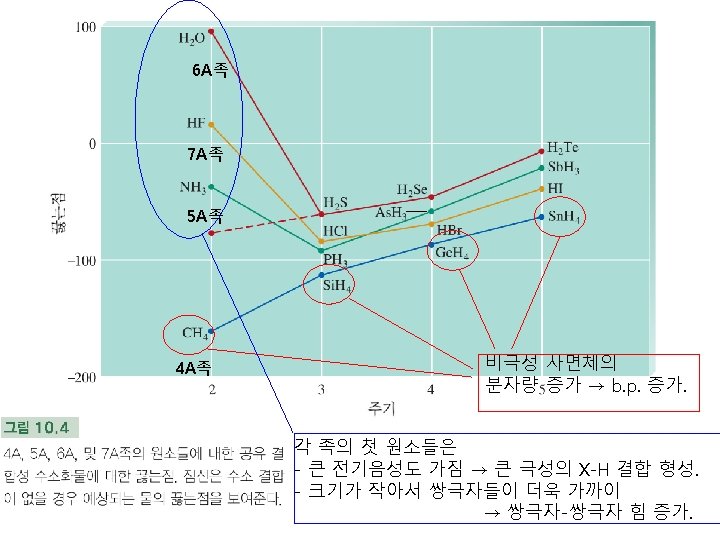
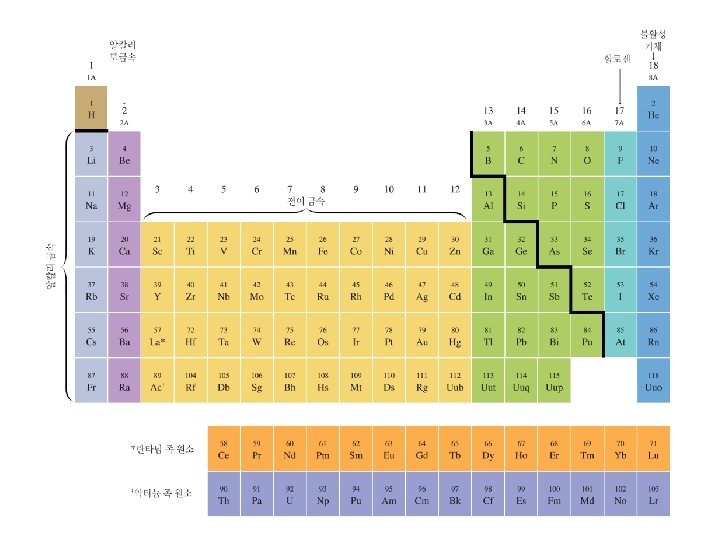
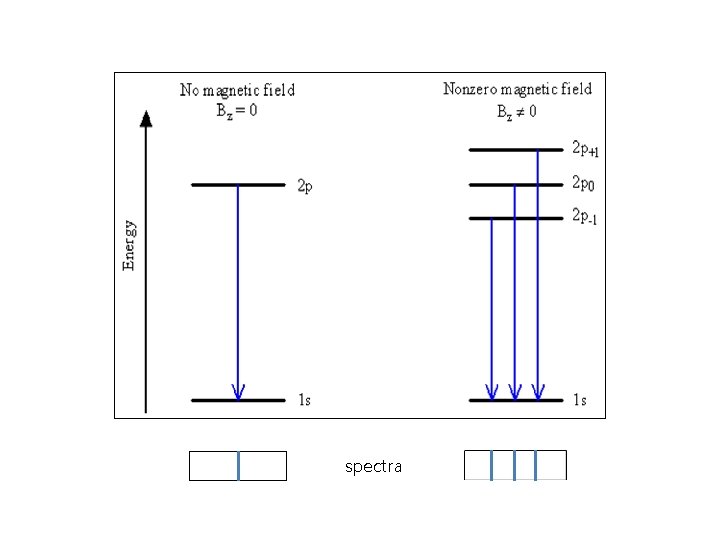
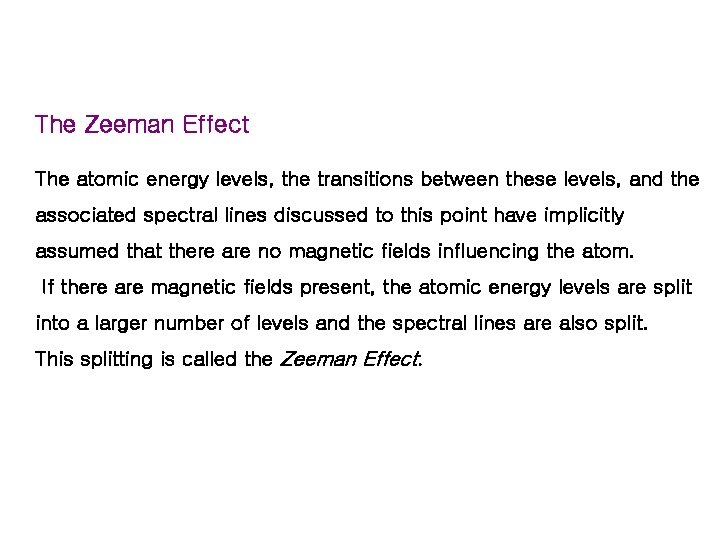
- Slides: 78
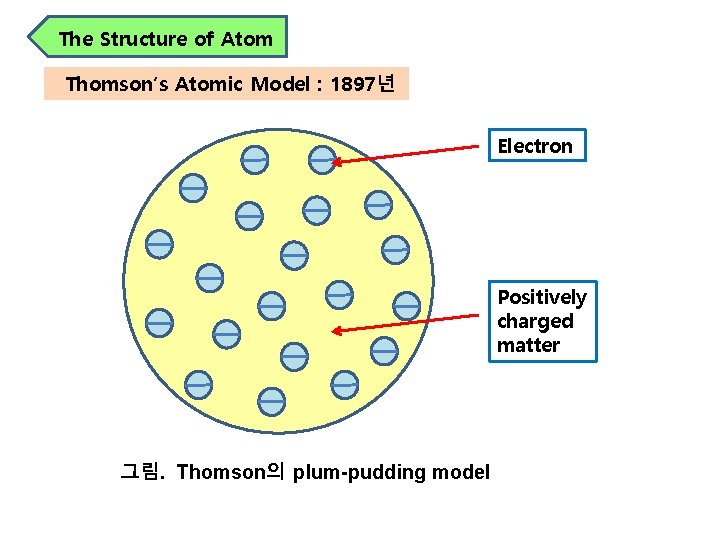
The Structure of Atom Thomson′s Atomic Model : 1897년 Electron Positively charged matter 그림. Thomson의 plum-pudding model
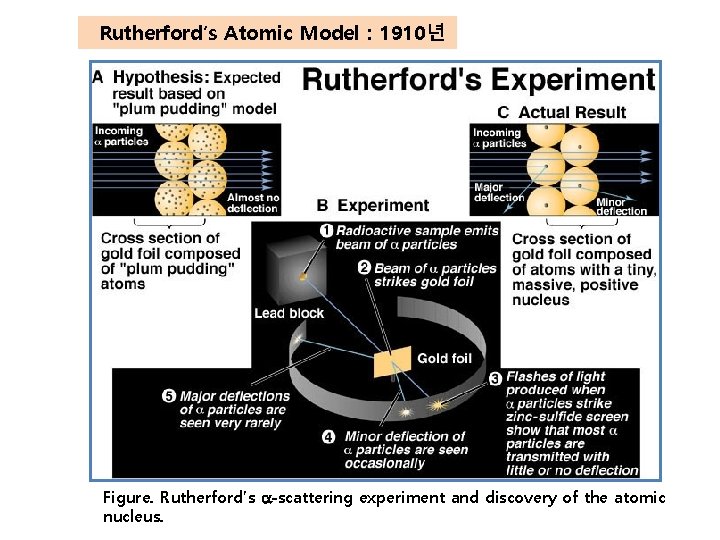
Rutherford′s Atomic Model : 1910년 Figure. Rutherford’s a-scattering experiment and discovery of the atomic nucleus.
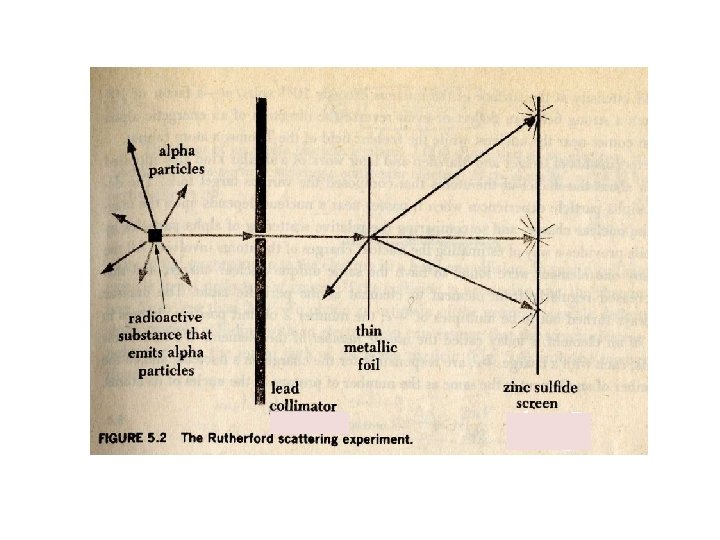
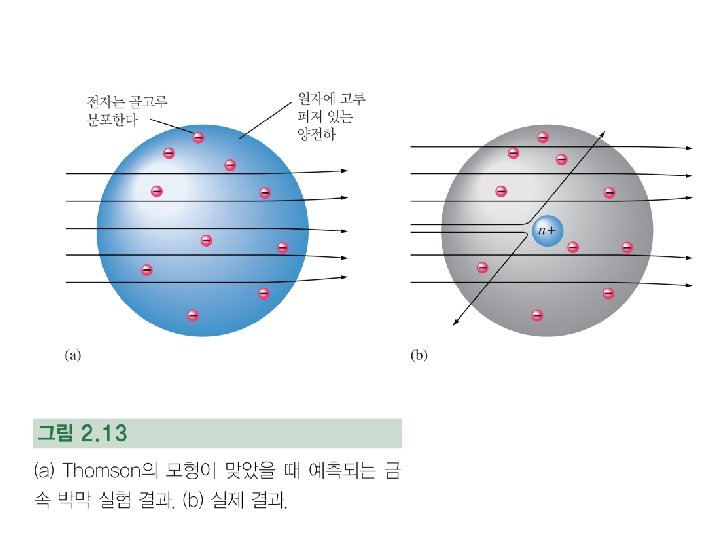
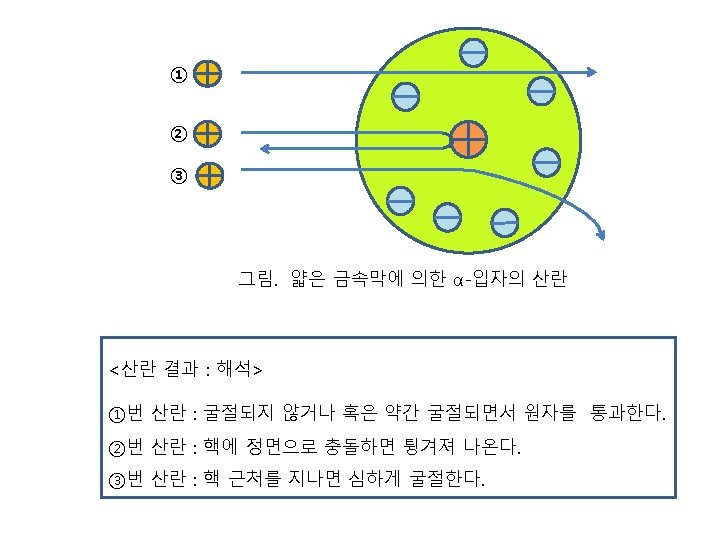
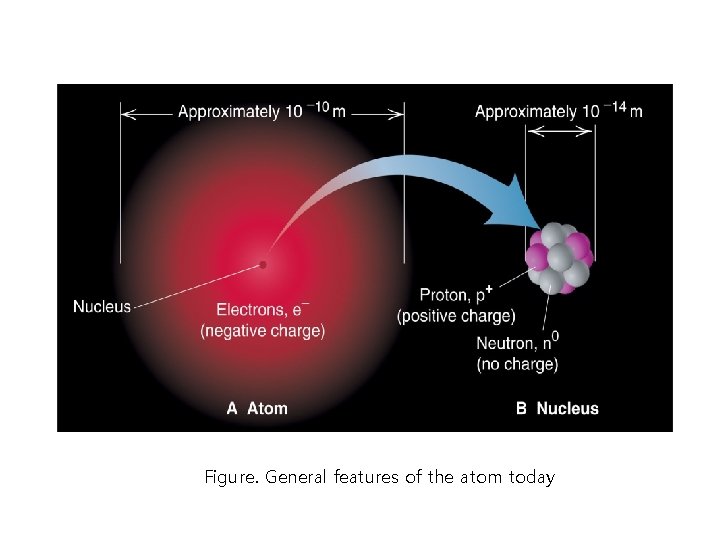
Figure. General features of the atom today
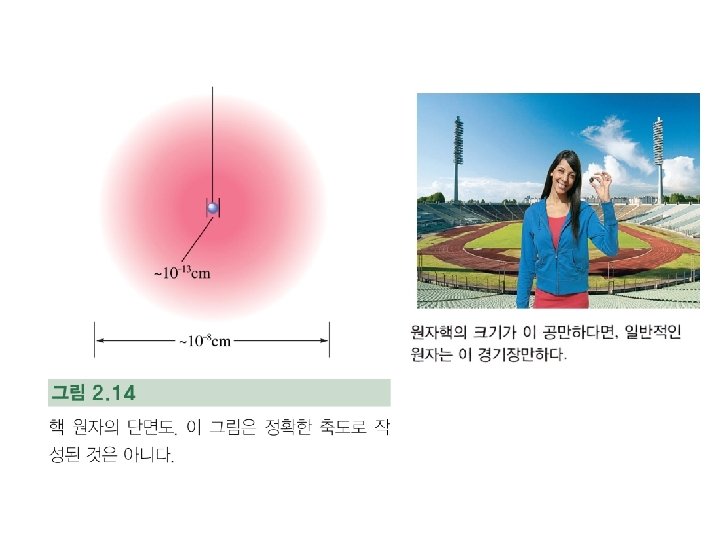
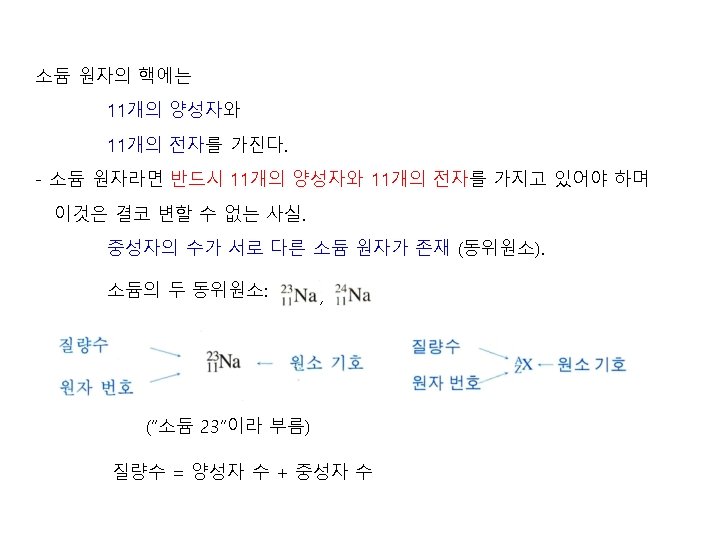
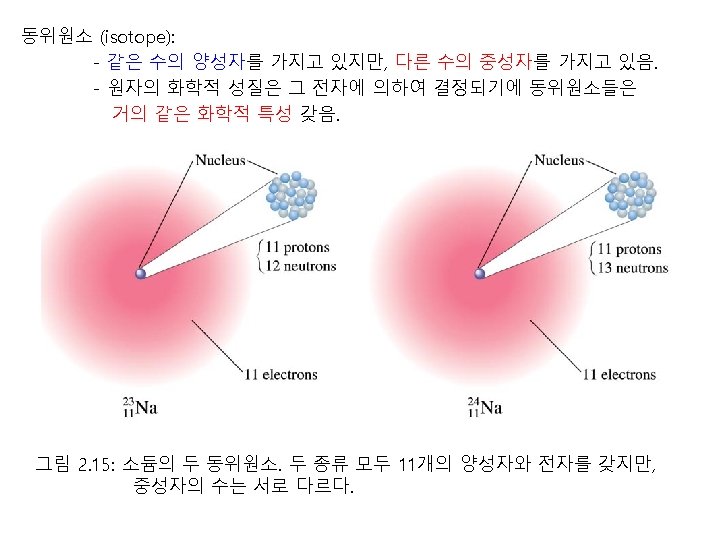
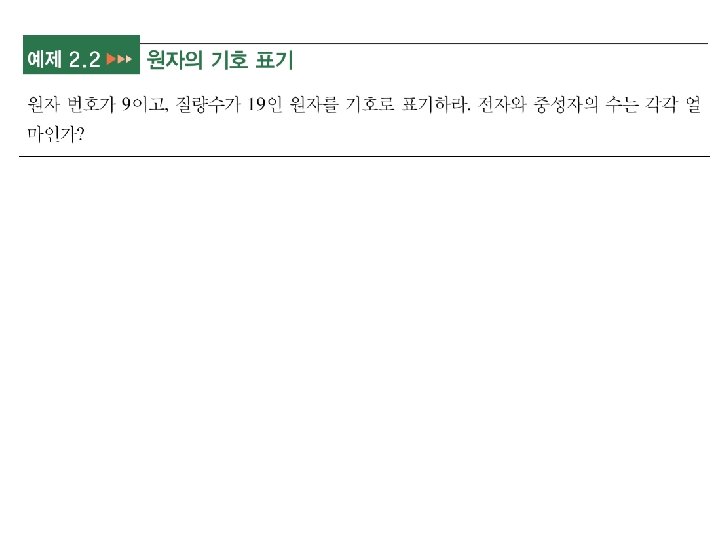
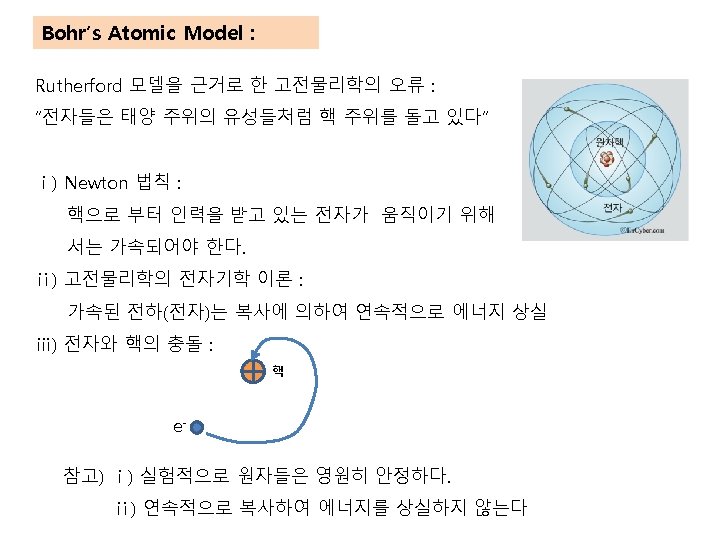
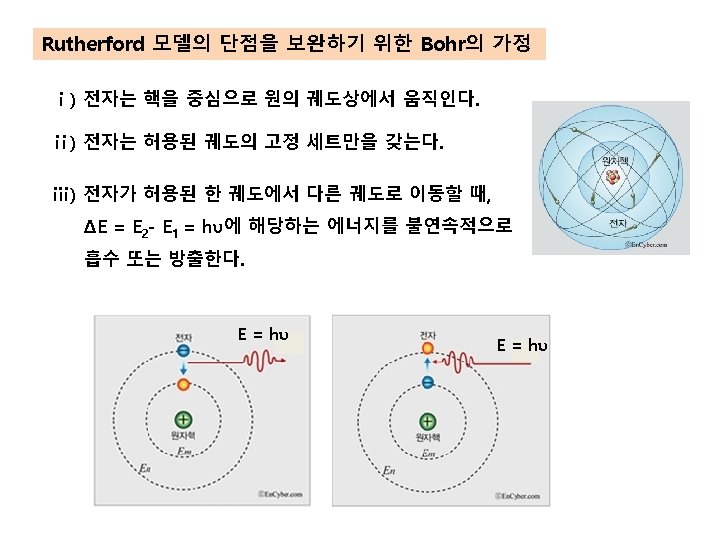
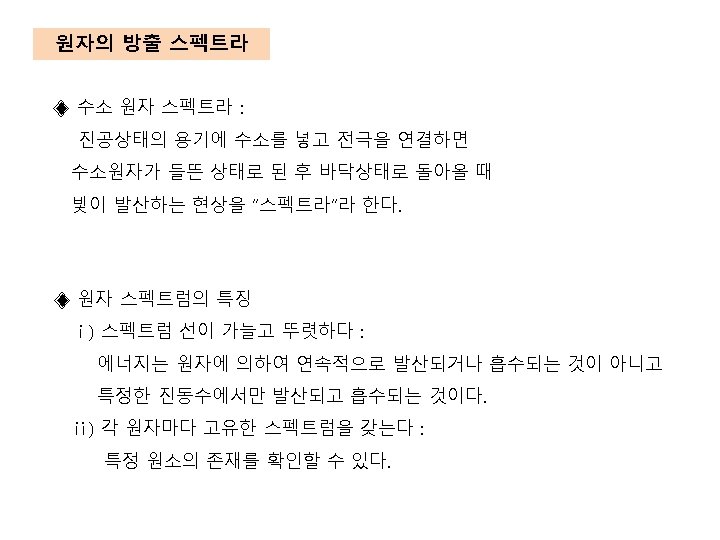
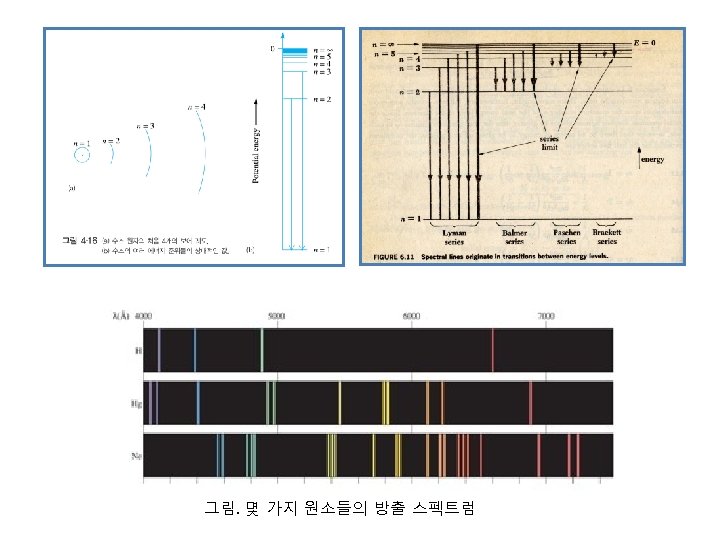
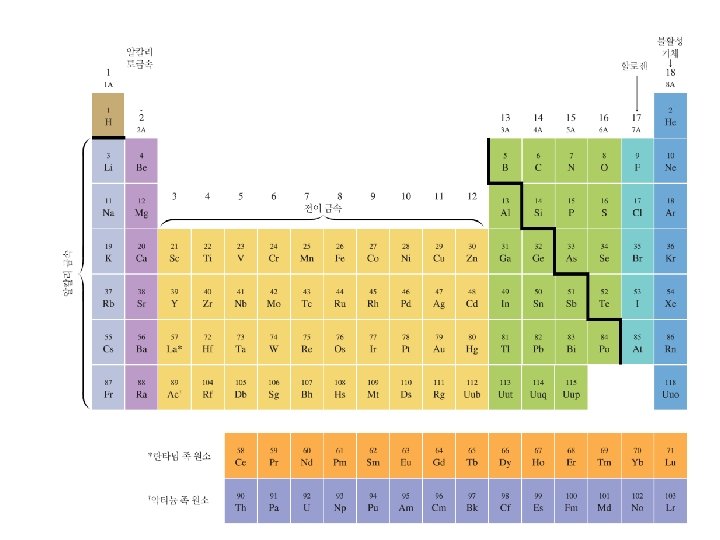
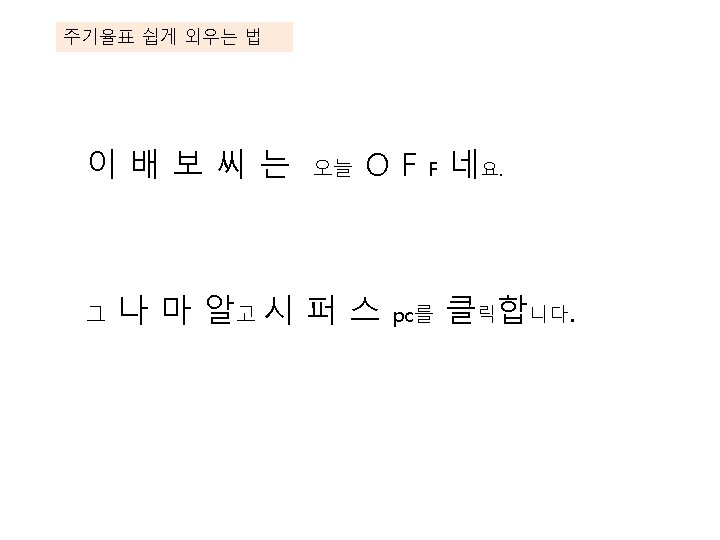
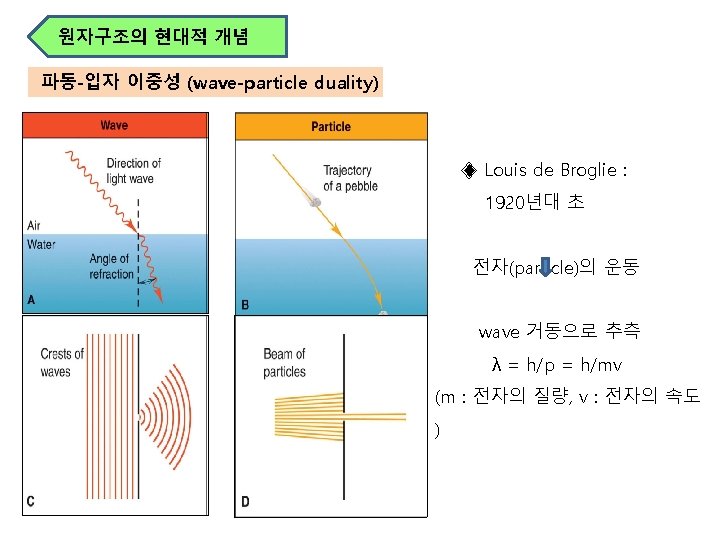
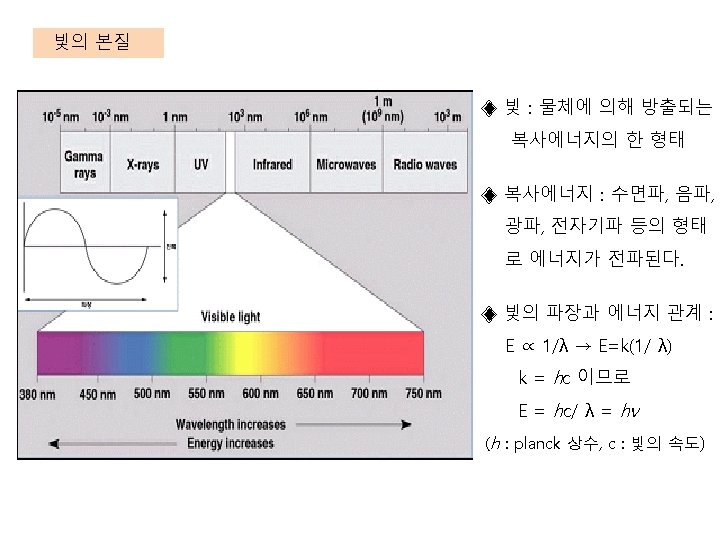
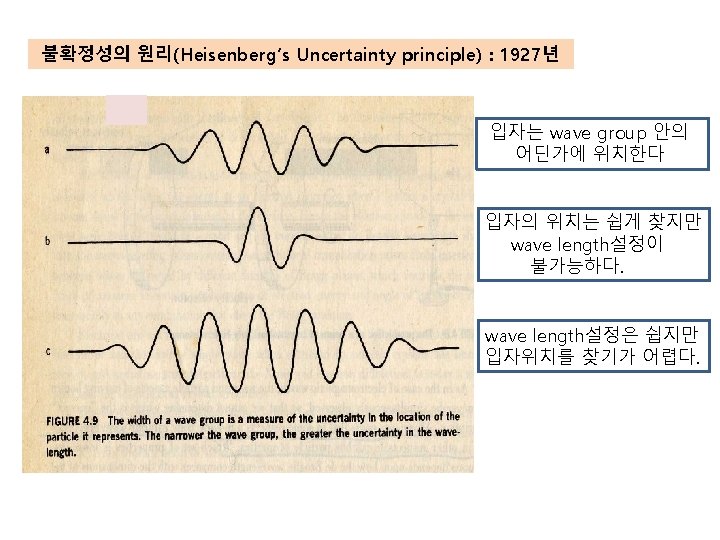
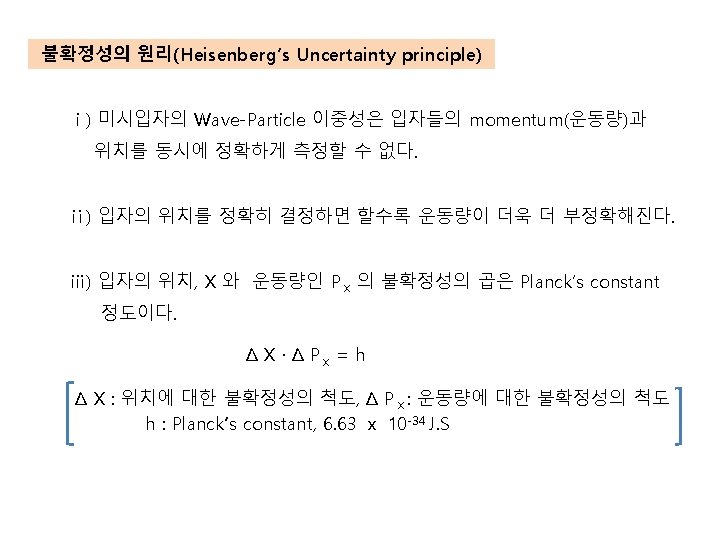
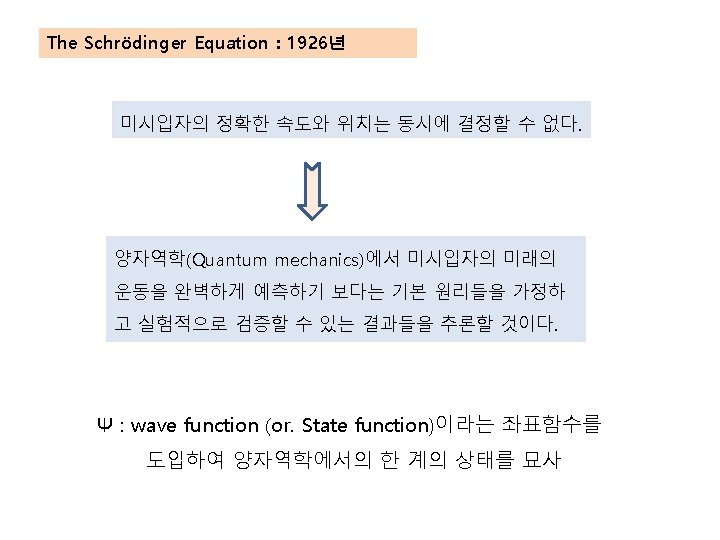
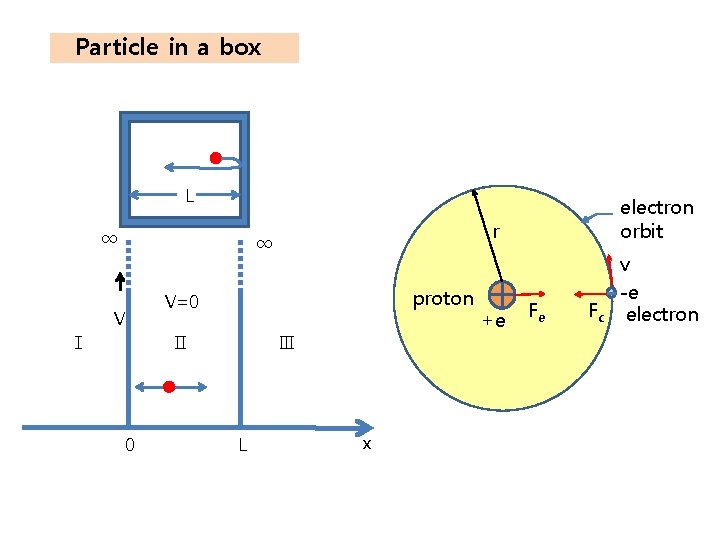
Particle in a box L ∞ Ⅰ r ∞ V 0 proton V=0 Ⅱ Ⅲ L electron orbit x +e Fe v -e Fc electron
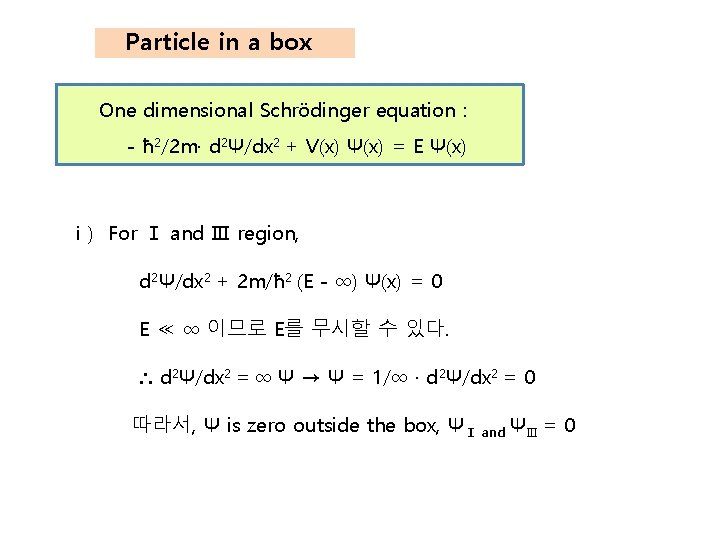
Particle in a box One dimensional Schrödinger equation : - ħ 2/2 m· d 2Ψ/dx 2 + V(x) Ψ(x) = E Ψ(x) ⅰ) For Ⅰ and Ⅲ region, d 2Ψ/dx 2 + 2 m/ħ 2 (E - ∞) Ψ(x) = 0 E ≪ ∞ 이므로 E를 무시할 수 있다. ∴ d 2Ψ/dx 2 = ∞ Ψ → Ψ = 1/∞ · d 2Ψ/dx 2 = 0 따라서, Ψ is zero outside the box, ΨⅠ and ΨⅢ = 0
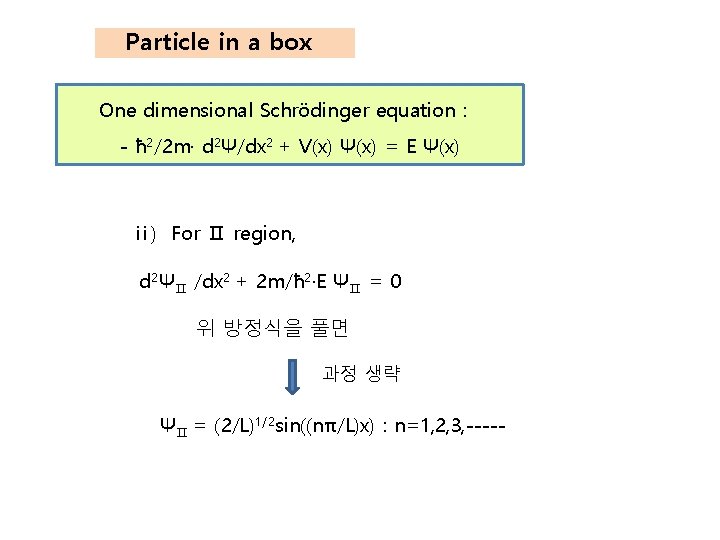
Particle in a box One dimensional Schrödinger equation : - ħ 2/2 m· d 2Ψ/dx 2 + V(x) Ψ(x) = E Ψ(x) ⅱ) For Ⅱ region, d 2ΨⅡ /dx 2 + 2 m/ħ 2·E ΨⅡ = 0 위 방정식을 풀면 과정 생략 ΨⅡ = (2/L)1/2 sin((nπ/L)x) : n=1, 2, 3, -----
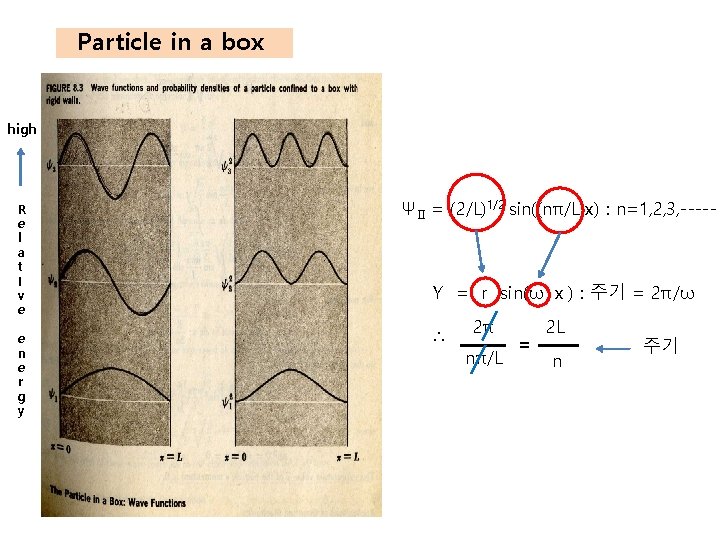
Particle in a box high R e l a t I v e e n e r g y ΨⅡ = (2/L)1/2 sin((nπ/L)x) : n=1, 2, 3, ----- Y = r sin(ω x) : 주기 = 2π/ω ∴ 2π nπ/L = 2 L n 주기
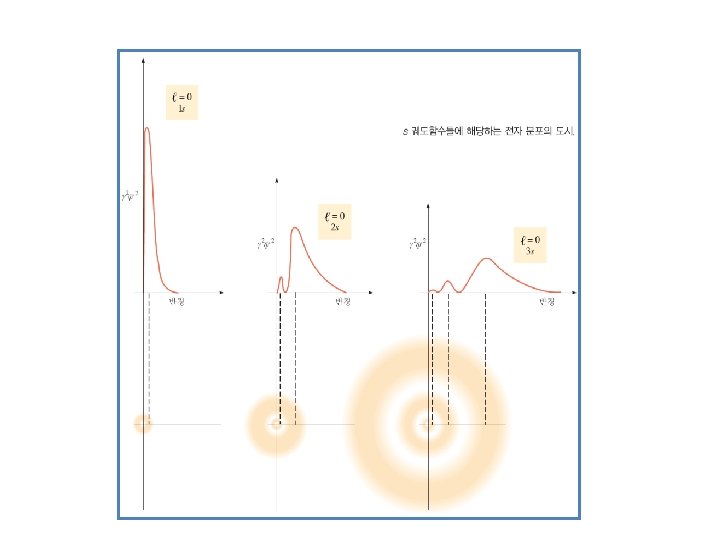
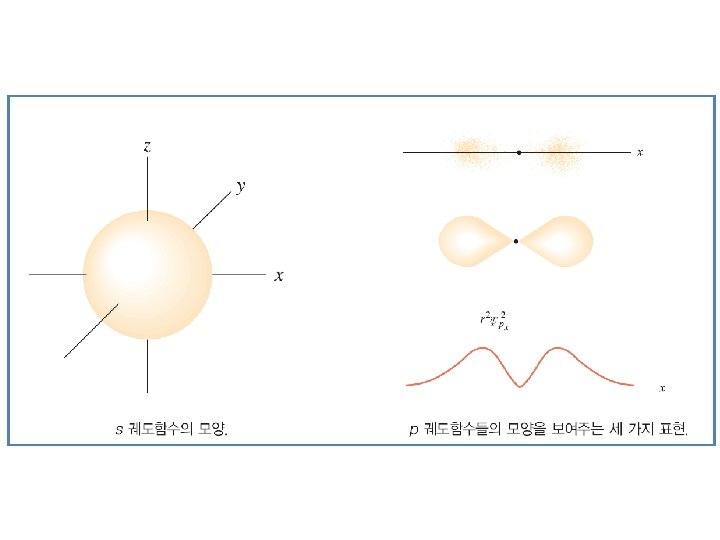
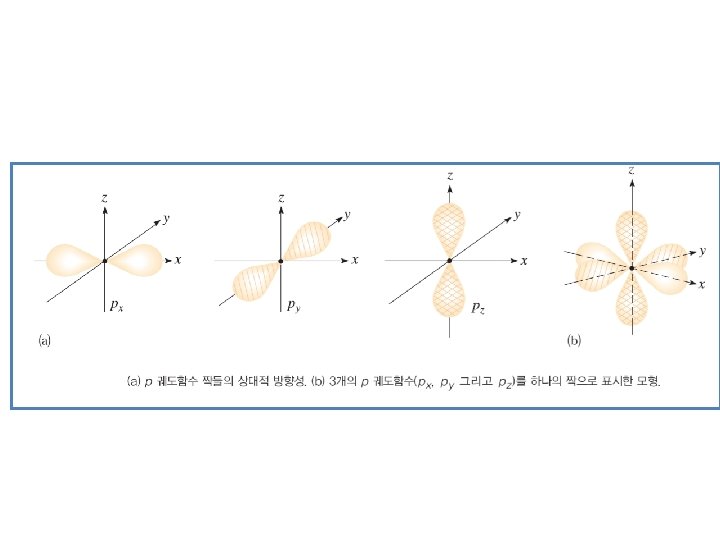
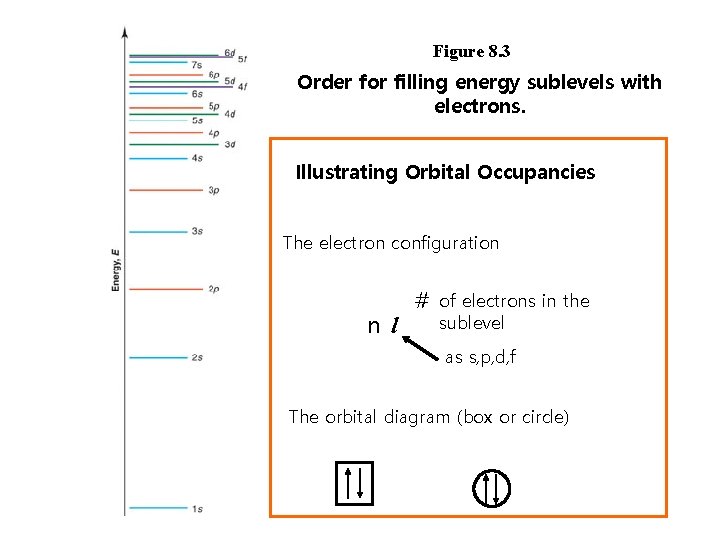
Figure 8. 3 Order for filling energy sublevels with electrons. Illustrating Orbital Occupancies The electron configuration n l # of electrons in the sublevel as s, p, d, f The orbital diagram (box or circle)
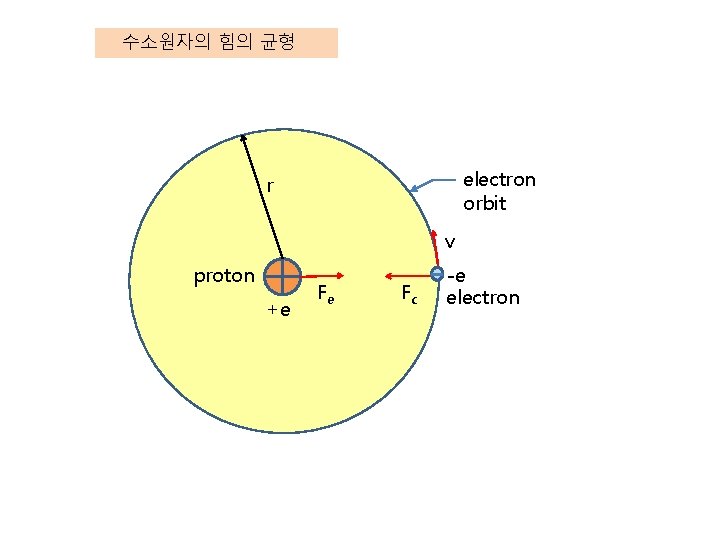
수소원자의 힘의 균형 electron orbit r v proton +e Fe Fc -e electron
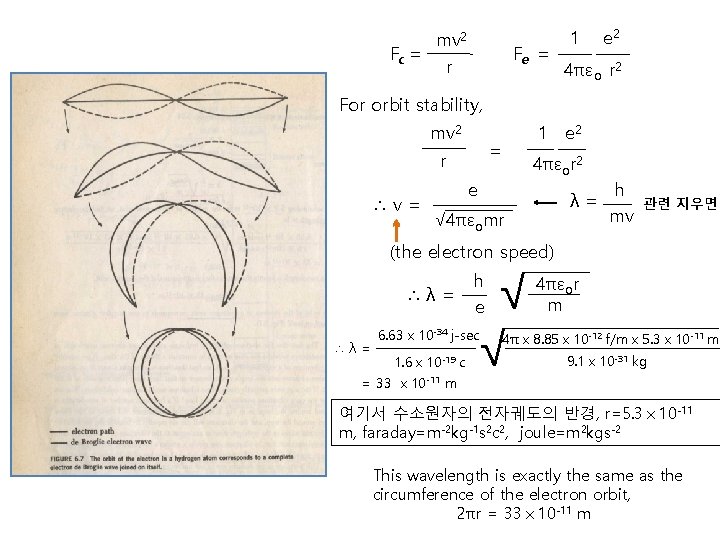
mv 2 Fc = r Fe = 1 e 2 4πεo r 2 For orbit stability, mv 2 = r ∴v= 1 e 2 4πεor 2 e λ= √ 4πεomr h mv 관련 지우면 (the electron speed) ∴λ= √ √ h e 6. 63x 10 -34 j-sec 1. 6x 10 -19 c = 33 x 10 -11 m 4πεor m 4πx 8. 85x 10 -12 f/mx 5. 3x 10 -11 m 9. 1x 10 -31 kg 여기서 수소원자의 전자궤도의 반경, r=5. 3x 10 -11 m, faraday=m-2 kg-1 s 2 c 2, joule=m 2 kgs-2 This wavelength is exactly the same as the circumference of the electron orbit, 2πr = 33x 10 -11 m
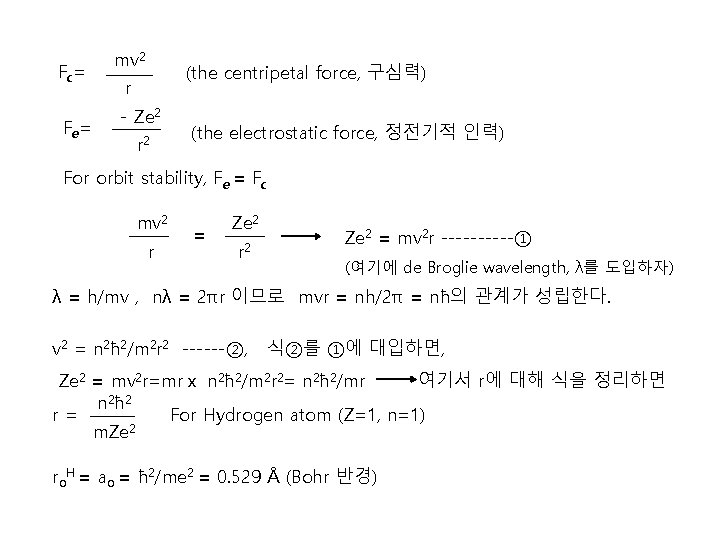
Fc= Fe= mv 2 (the centripetal force, 구심력) r - Ze 2 r 2 (the electrostatic force, 정전기적 인력) For orbit stability, Fe = Fc mv 2 r = Ze 2 r 2 Ze 2 = mv 2 r -----① (여기에 de Broglie wavelength, λ를 도입하자) λ = h/mv , nλ = 2πr 이므로 mvr = nh/2π = nħ의 관계가 성립한다. v 2 = n 2ħ 2/m 2 r 2 ------②, 식②를 ①에 대입하면, 여기서 r에 대해 식을 정리하면 Ze 2 = mv 2 r=mrx n 2ħ 2/m 2 r 2= n 2ħ 2/mr n 2ħ 2 r= For Hydrogen atom (Z=1, n=1) 2 m. Ze ro. H = ao = ħ 2/me 2 = 0. 529 Å (Bohr 반경)
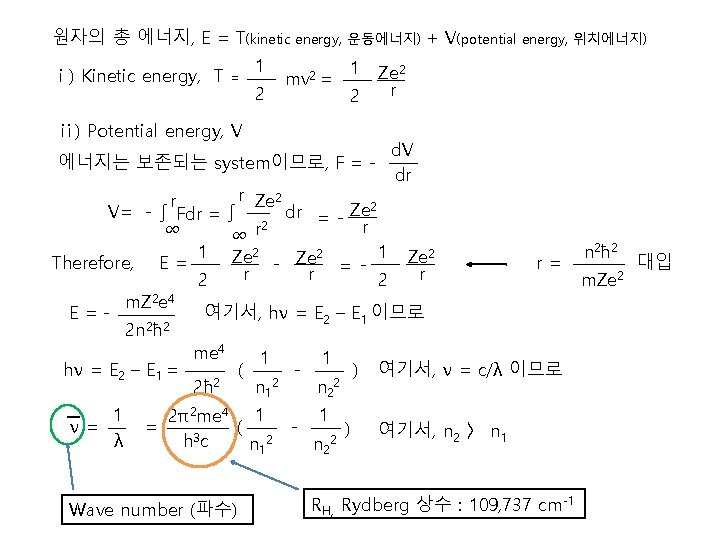
원자의 총 에너지, E = T(kinetic energy, 운동에너지) + V(potential energy, 위치에너지) ⅰ) Kinetic energy, T = 1 2 mv 2 = 1 2 ⅱ) Potential energy, V 에너지는 보존되는 system이므로, F = - Ze 2 r d. V dr r Ze 2 r dr = - Ze 2 V= - ∫ Fdr = ∫ 2 r ∞ ∞ r 1 Ze 2 2 Therefore, E = r= - Ze = r r r 2 2 m. Z 2 e 4 E=여기서, hν = E 2 – E 1 이므로 2 2 2 n ħ me 4 1 1 hν = E 2 – E 1 = ( ) 여기서, ν = c/λ 이므로 2 2ħ n 1 n 2 1 ν= λ 2π2 me 4 1 = ( h 3 c n 12 Wave number (파수) - 1 n 22 ) 여기서, n 2 〉 n 1 RH, Rydberg 상수 : 109, 737 cm-1 n 2ħ 2 m. Ze 2 대입
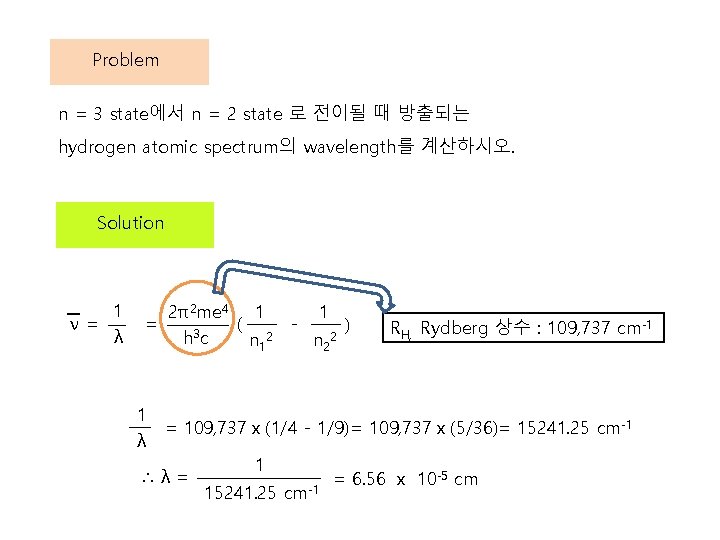
Problem n = 3 state에서 n = 2 state 로 전이될 때 방출되는 hydrogen atomic spectrum의 wavelength를 계산하시오. Solution 1 ν= λ 2π2 me 4 1 = ( h 3 c n 12 1 λ - 1 n 22 ) RH, Rydberg 상수 : 109, 737 cm-1 = 109, 737x(1/4 - 1/9)= 109, 737x(5/36)= 15241. 25 cm-1 ∴λ= 1 15241. 25 cm-1 = 6. 56 x 10 -5 cm
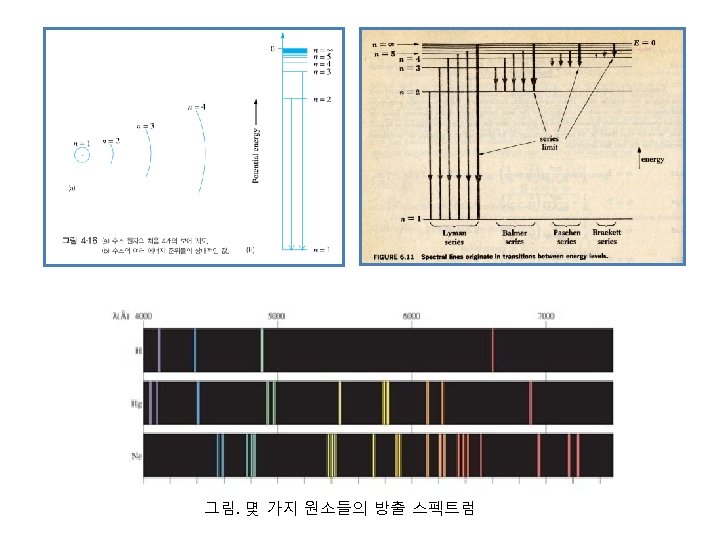
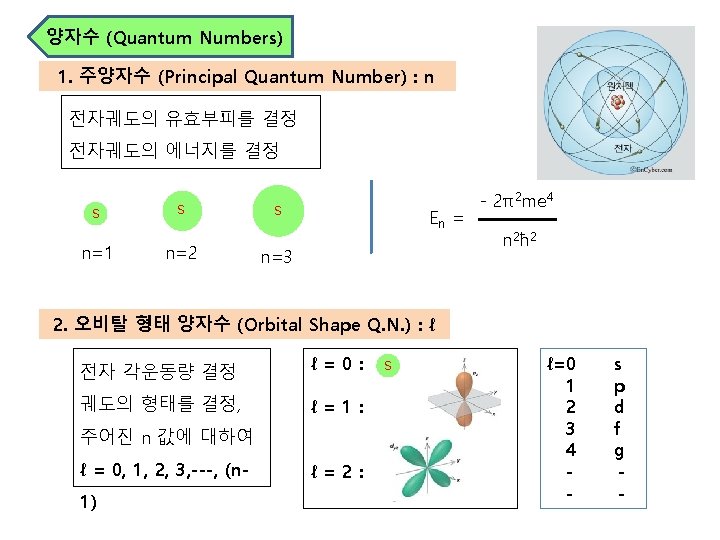
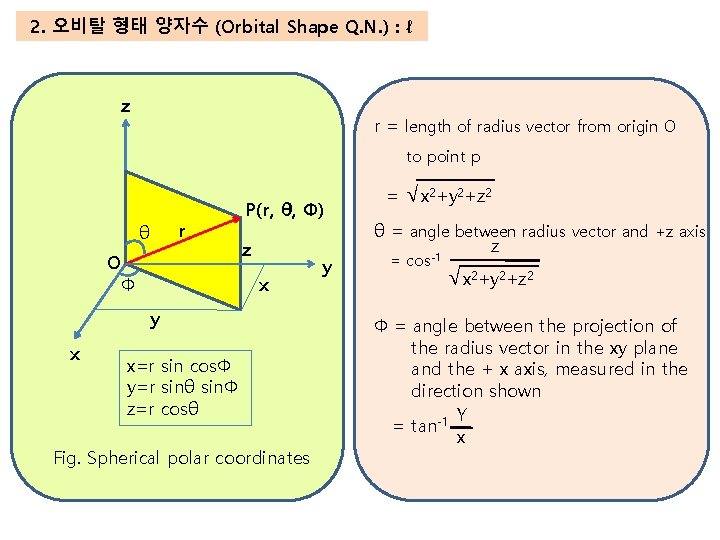
2. 오비탈 형태 양자수 (Orbital Shape Q. N. ) : ℓ z r = length of radius vector from origin O to point p r θ O Φ P(r, θ, Φ) z x y x x=r sin cosΦ y=r sinθ sinΦ z=r cosθ Fig. Spherical polar coordinates y = √x 2+y 2+z 2 θ = angle between radius vector and +z axis z = cos-1 √x 2+y 2+z 2 Φ = angle between the projection of the radius vector in the xy plane and the + x axis, measured in the direction shown Y = tan-1 x
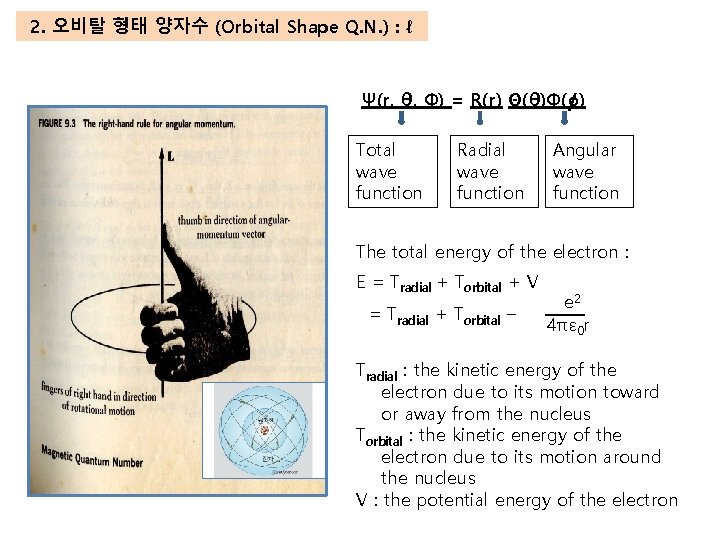
2. 오비탈 형태 양자수 (Orbital Shape Q. N. ) : ℓ Ψ(r, θ, Φ) = R(r) Θ(θ)Φ(o) Total wave function Radial wave function Angular wave function The total energy of the electron : E = Tradial + Torbital + V = Tradial + Torbital – e 2 4πε 0 r Tradial : the kinetic energy of the electron due to its motion toward or away from the nucleus Torbital : the kinetic energy of the electron due to its motion around the nucleus V : the potential energy of the electron
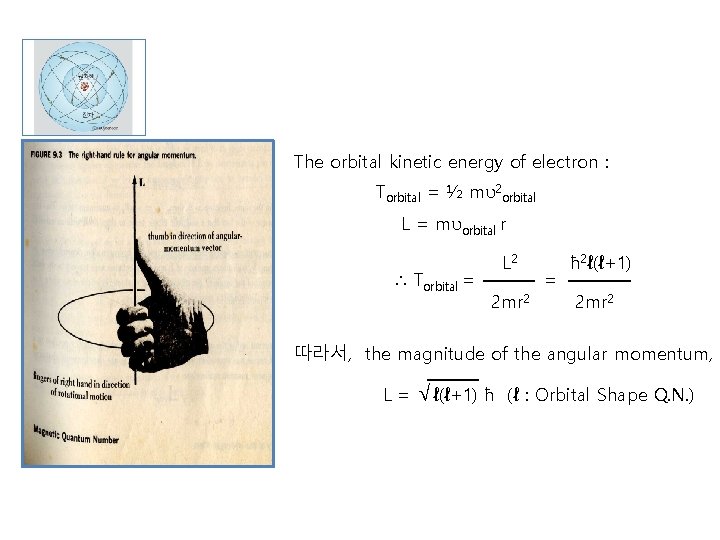
The orbital kinetic energy of electron : Torbital = ½ mυ2 orbital L = mυorbital r ∴ Torbital = L 2 2 mr 2 = ħ 2ℓ(ℓ+1) 2 mr 2 따라서, the magnitude of the angular momentum, L= √ℓ(ℓ+1) ħ (ℓ : Orbital Shape Q. N. )
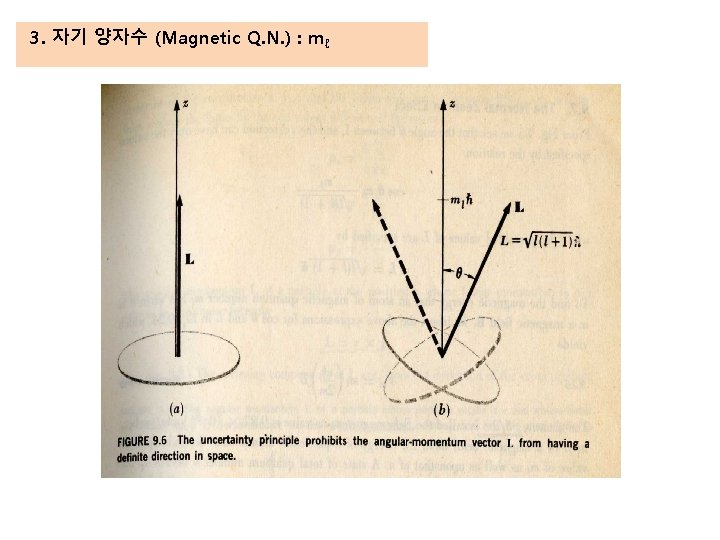
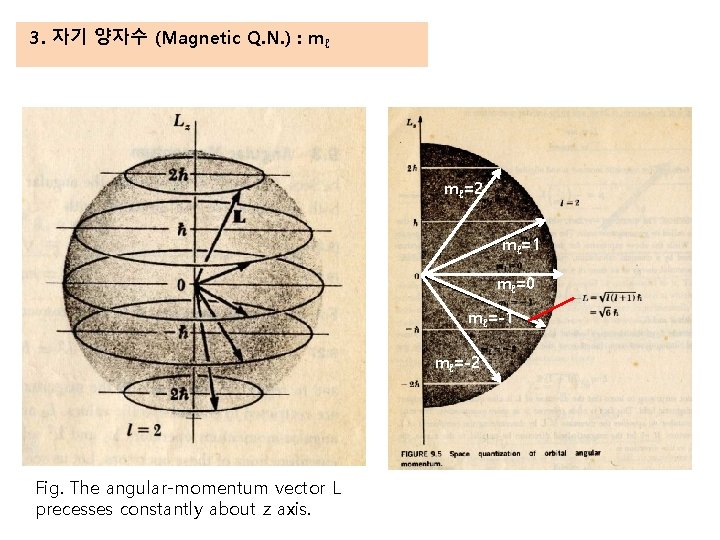
3. 자기 양자수 (Magnetic Q. N. ) : mℓ mℓ=2 mℓ=1 mℓ=0 mℓ=-1 mℓ=-2 Fig. The angular-momentum vector L precesses constantly about z axis.
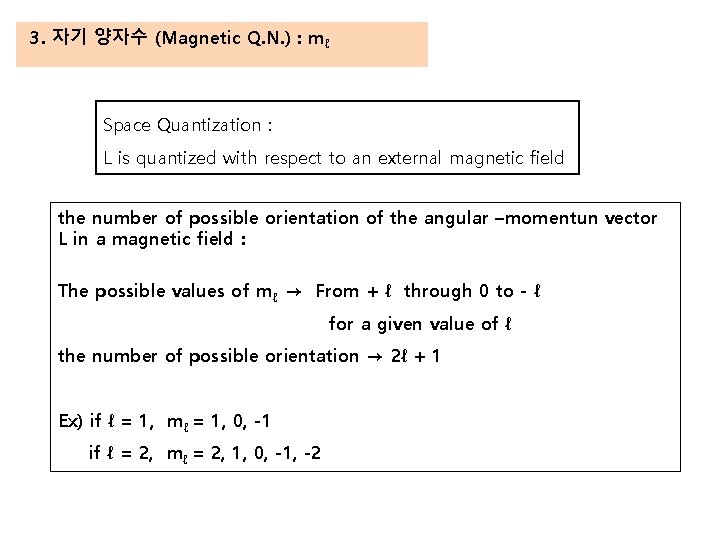
3. 자기 양자수 (Magnetic Q. N. ) : mℓ Space Quantization : L is quantized with respect to an external magnetic field the number of possible orientation of the angular –momentun vector L in a magnetic field : The possible values of mℓ → From + ℓ through 0 to - ℓ for a given value of ℓ the number of possible orientation → 2ℓ + 1 Ex) if ℓ = 1, mℓ = 1, 0, -1 if ℓ = 2, mℓ = 2, 1, 0, -1, -2
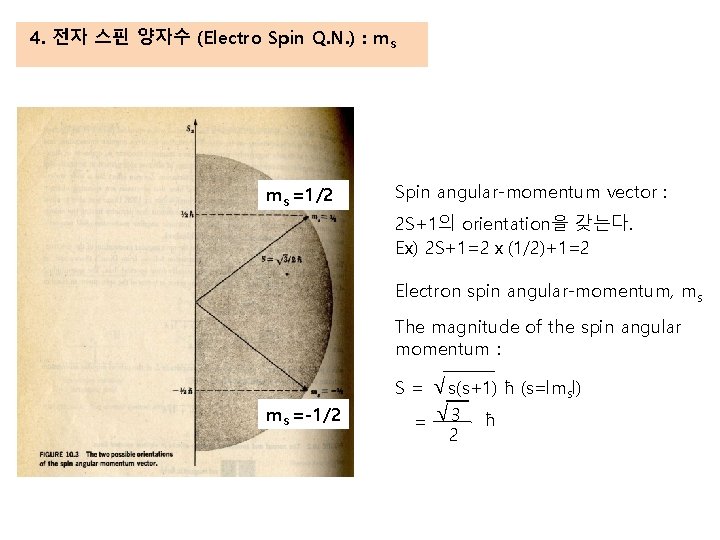
4. 전자 스핀 양자수 (Electro Spin Q. N. ) : ms ms =1/2 Spin angular-momentum vector : 2 S+1의 orientation을 갖는다. Ex) 2 S+1=2x(1/2)+1=2 Electron spin angular-momentum, ms The magnitude of the spin angular momentum : √s(s+1) ħ (s=lmsl) = √ 3 ħ S= ms =-1/2 2
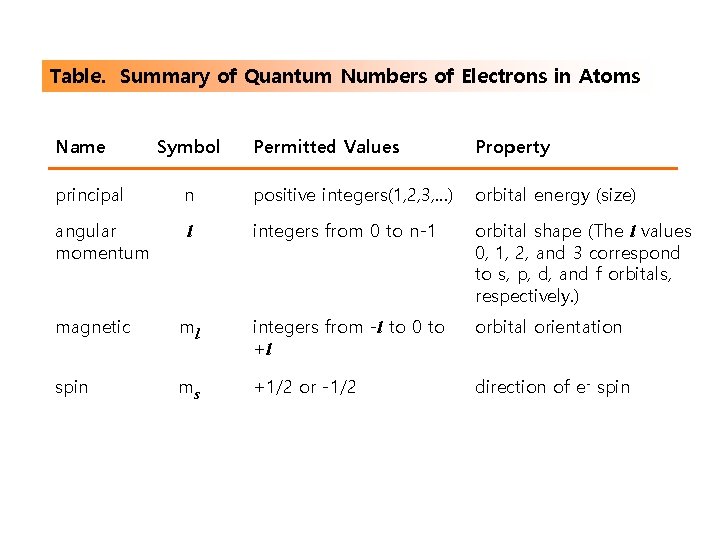
Table. Summary of Quantum Numbers of Electrons in Atoms Name Symbol Permitted Values Property principal n positive integers(1, 2, 3, …) orbital energy (size) angular momentum l integers from 0 to n-1 orbital shape (The l values 0, 1, 2, and 3 correspond to s, p, d, and f orbitals, respectively. ) magnetic ml integers from -l to 0 to +l orbital orientation spin ms +1/2 or -1/2 direction of e- spin
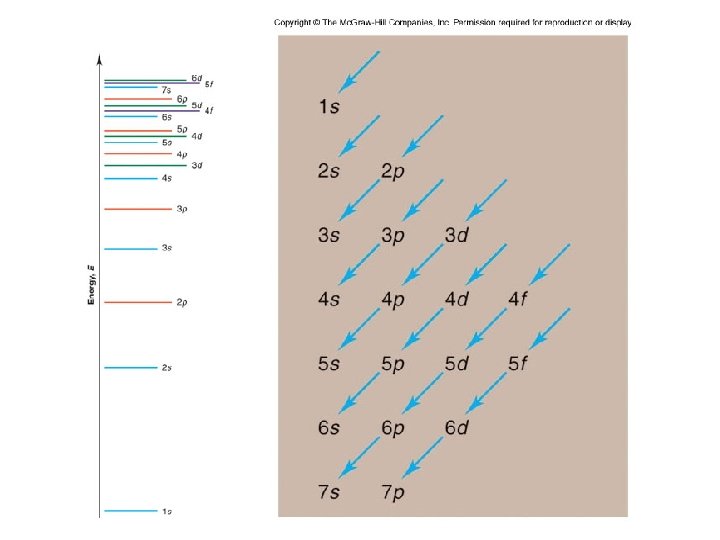
Figure 8. 7
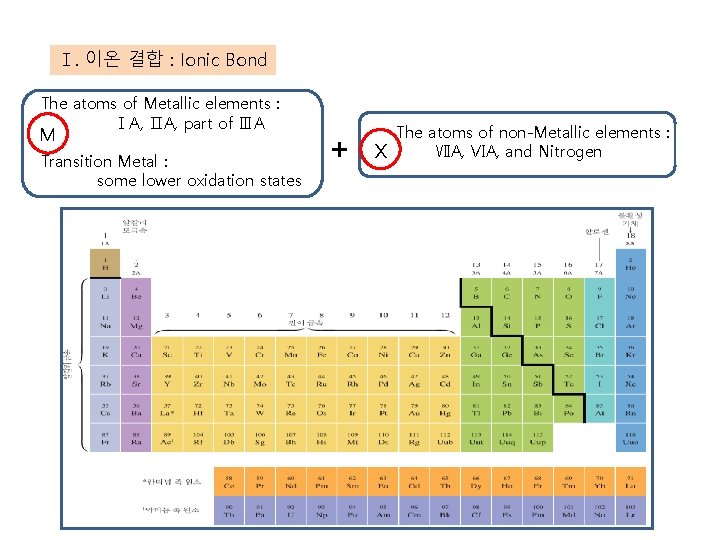
Ⅰ. 이온 결합 : Ionic Bond The atoms of Metallic elements : ⅠA, ⅡA, part of ⅢA M Transition Metal : some lower oxidation states + X The atoms of non-Metallic elements : ⅦA, ⅥA, and Nitrogen
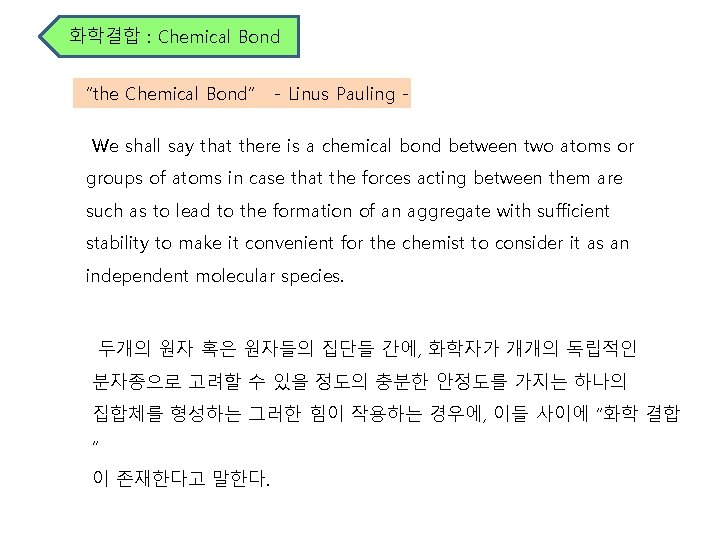
화학결합 : Chemical Bond “the Chemical Bond” - Linus Pauling - We shall say that there is a chemical bond between two atoms or groups of atoms in case that the forces acting between them are such as to lead to the formation of an aggregate with sufficient stability to make it convenient for the chemist to consider it as an independent molecular species. 두개의 원자 혹은 원자들의 집단들 간에, 화학자가 개개의 독립적인 분자종으로 고려할 수 있을 정도의 충분한 안정도를 가지는 하나의 집합체를 형성하는 그러한 힘이 작용하는 경우에, 이들 사이에 “화학 결합 ” 이 존재한다고 말한다.
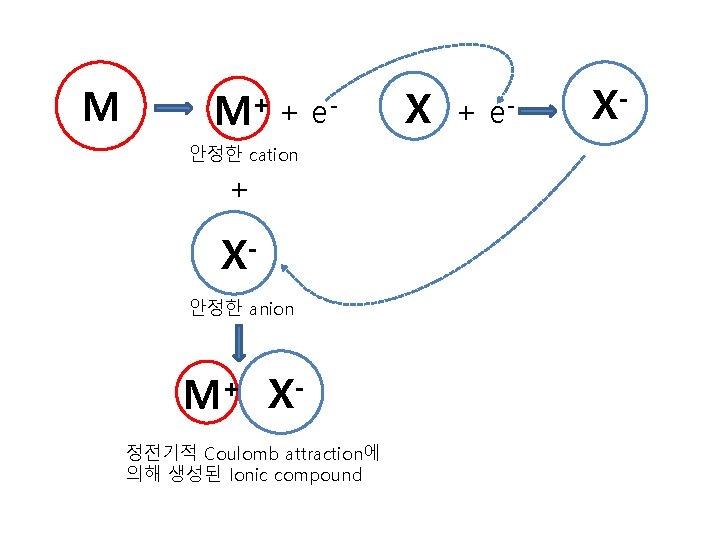
M M+ + e- 안정한 cation + X안정한 anion M+ X정전기적 Coulomb attraction에 의해 생성된 Ionic compound X + e- X-
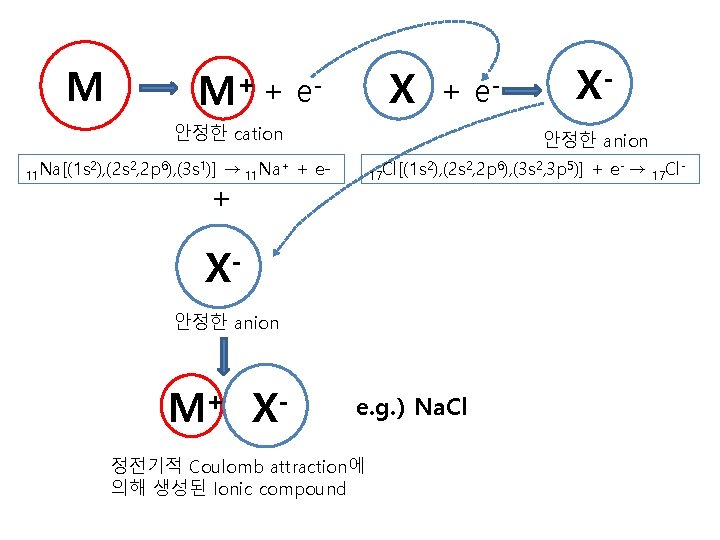
M M+ + X e- + 안정한 cation 11 Na[(1 s 2), (2 s 2, 2 p 6), (3 s 1)] X안정한 anion → 11 Na+ + e- 17 Cl[(1 s + 2), (2 s 2, 2 p 6), (3 s 2, 3 p 5)] X안정한 anion M+ X- e- e. g. ) Na. Cl 정전기적 Coulomb attraction에 의해 생성된 Ionic compound + e- → 17 Cl -
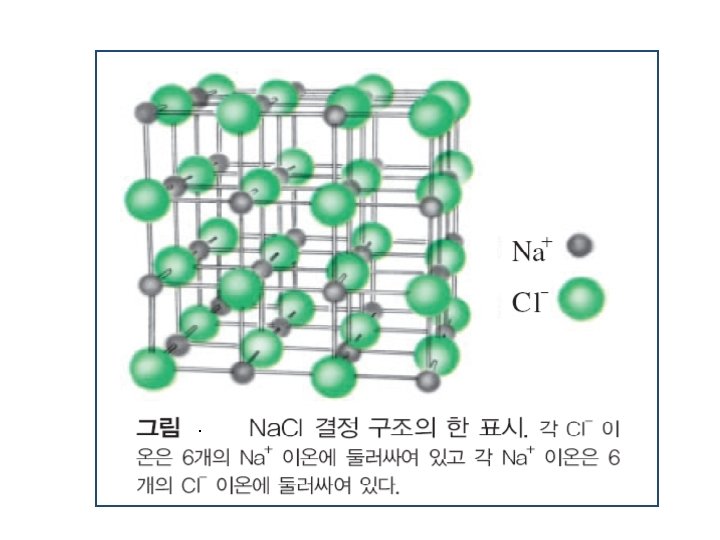
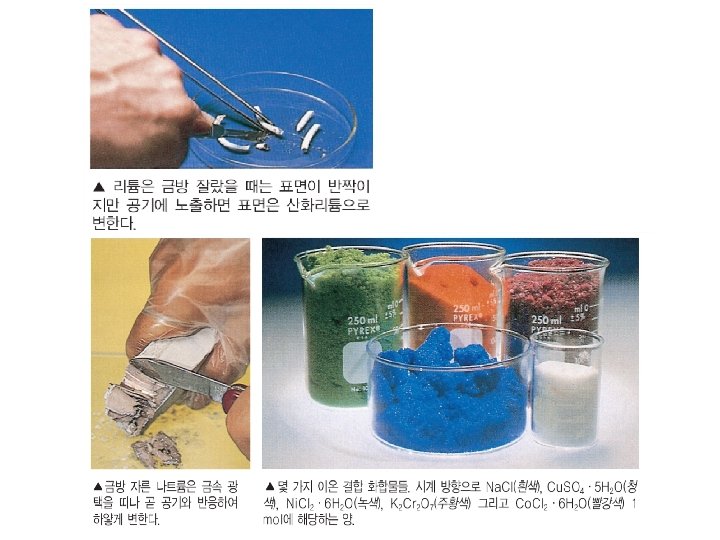
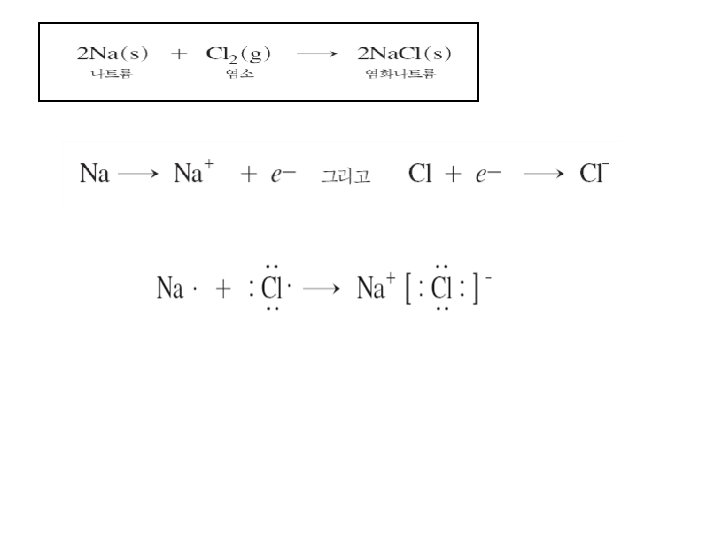
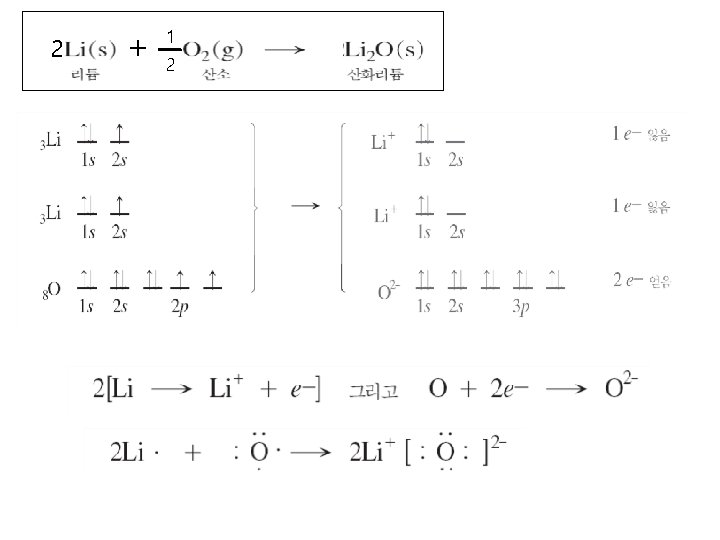
2 + 1 2
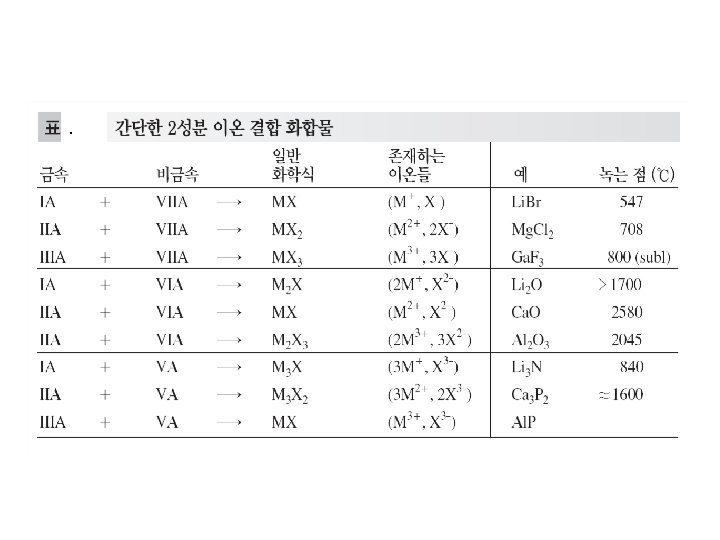
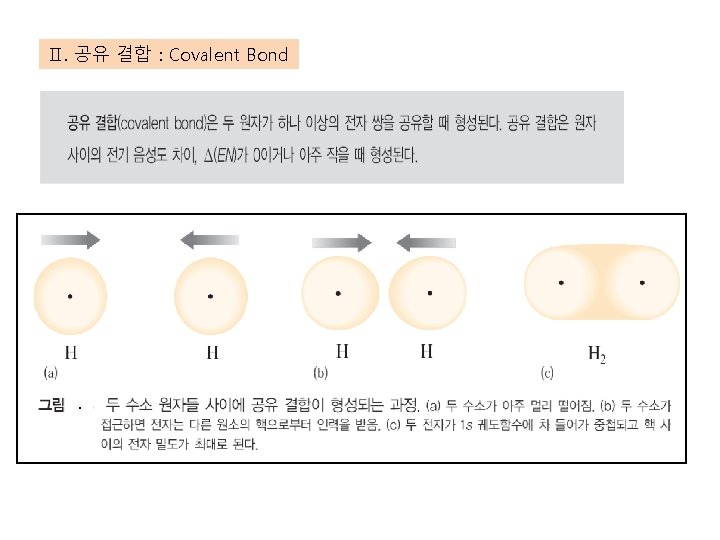
Ⅱ. 공유 결합 : Covalent Bond .
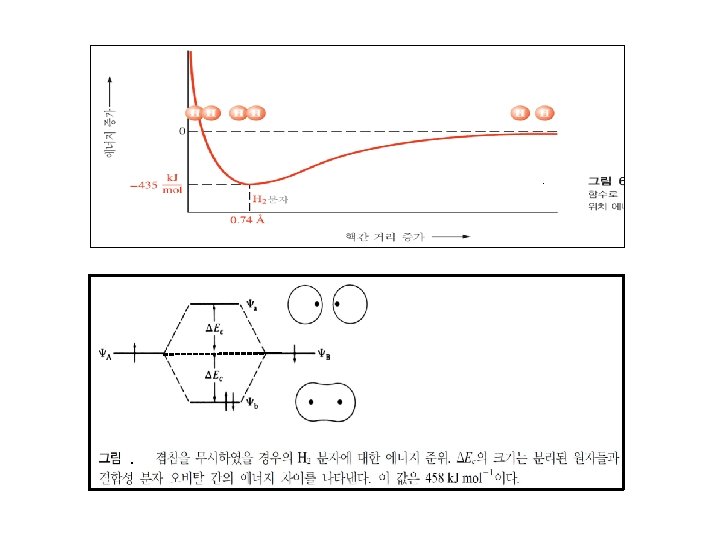
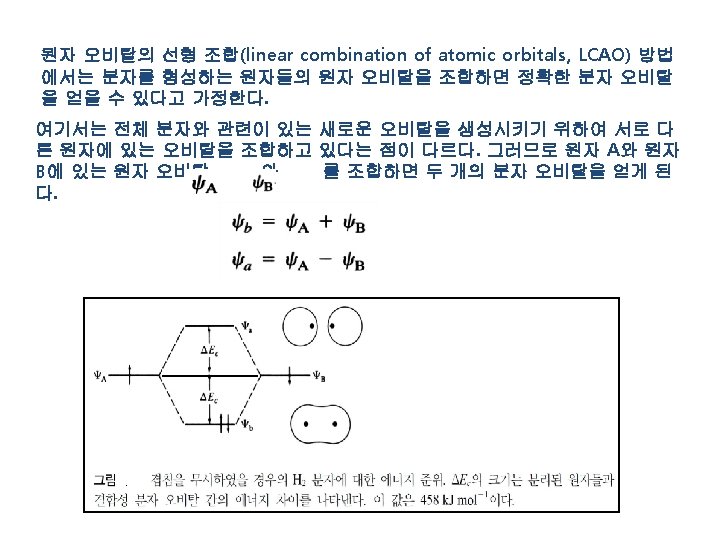
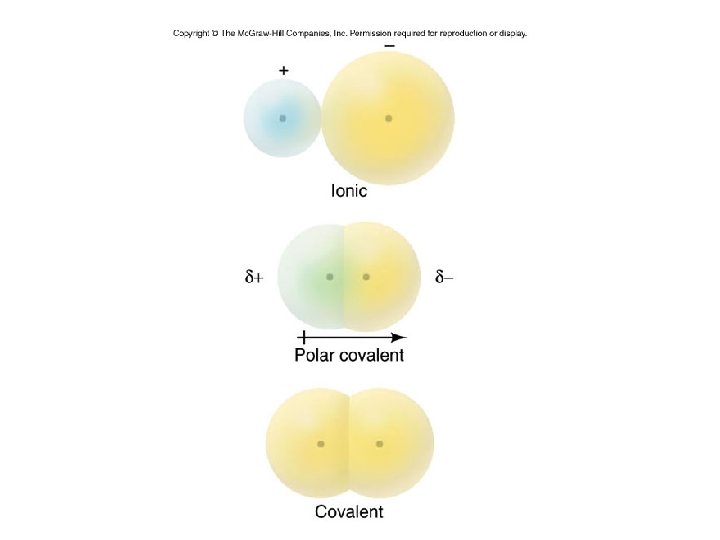
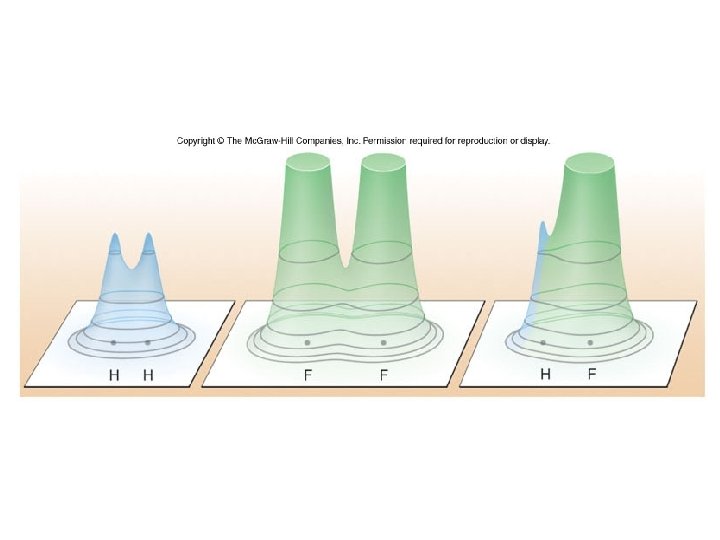
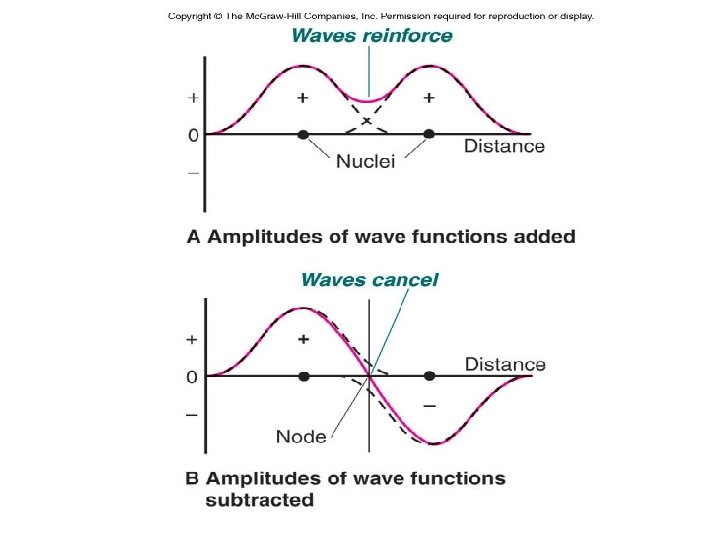
Figure 11. 13
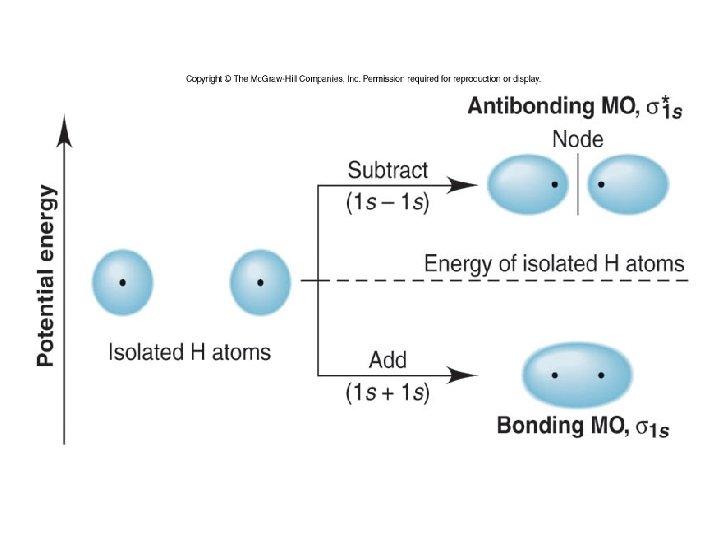
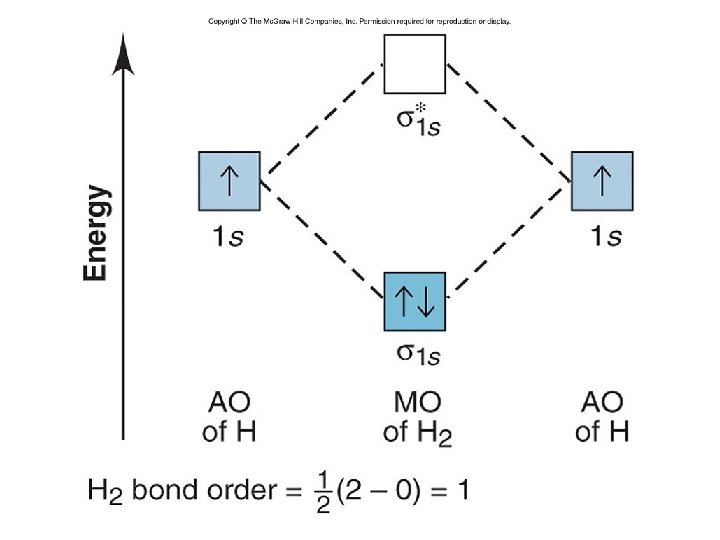
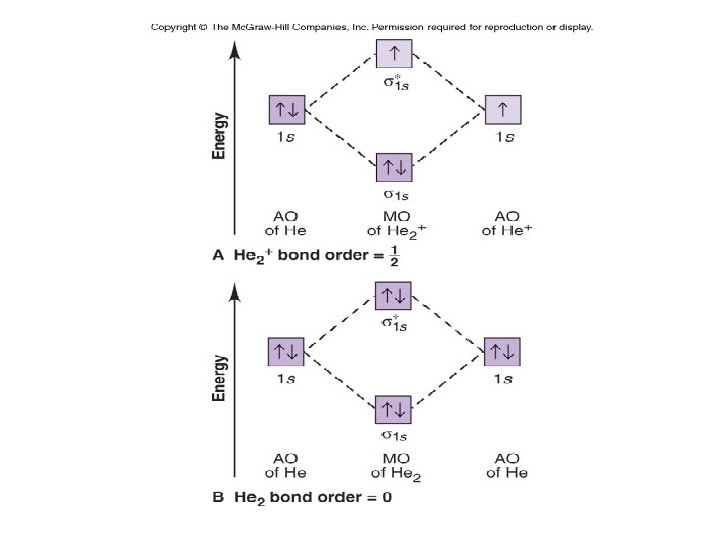
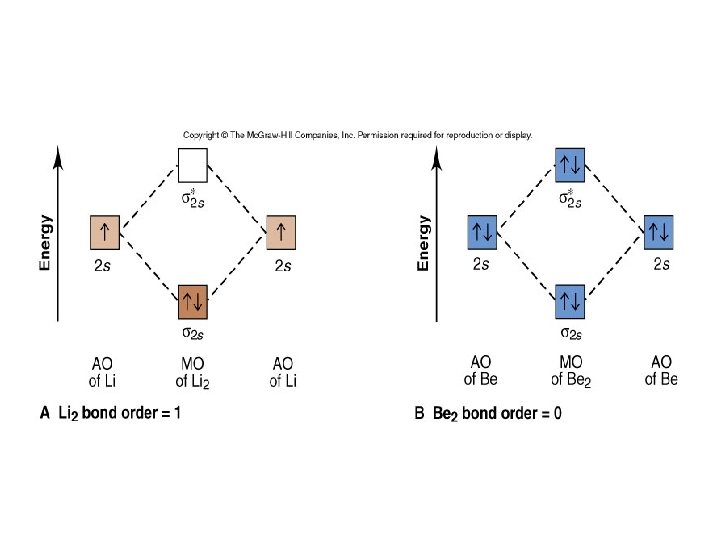
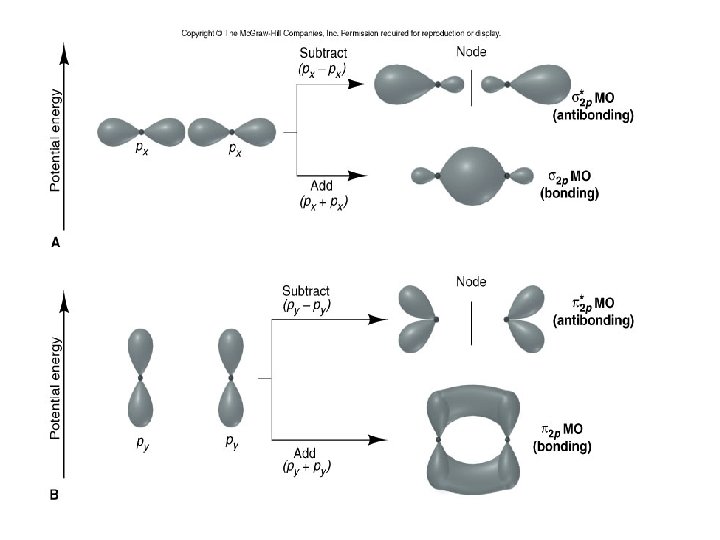
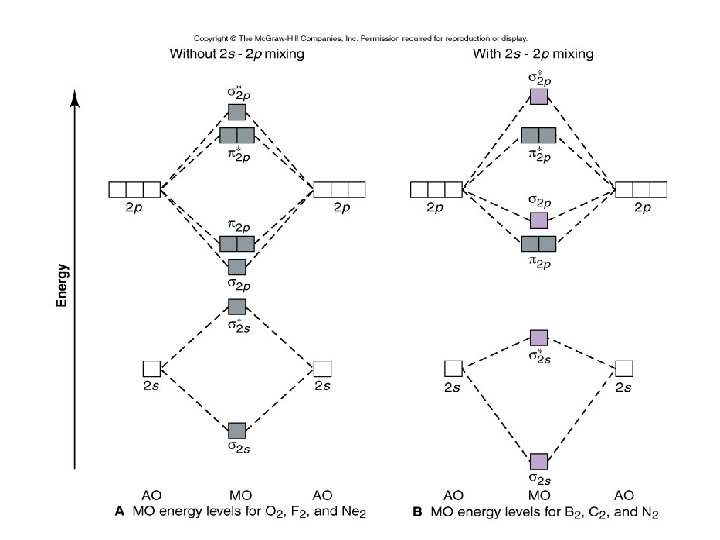
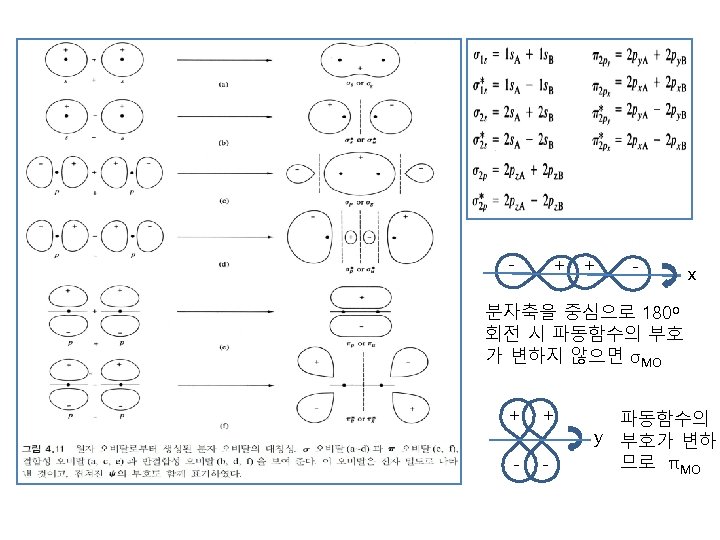
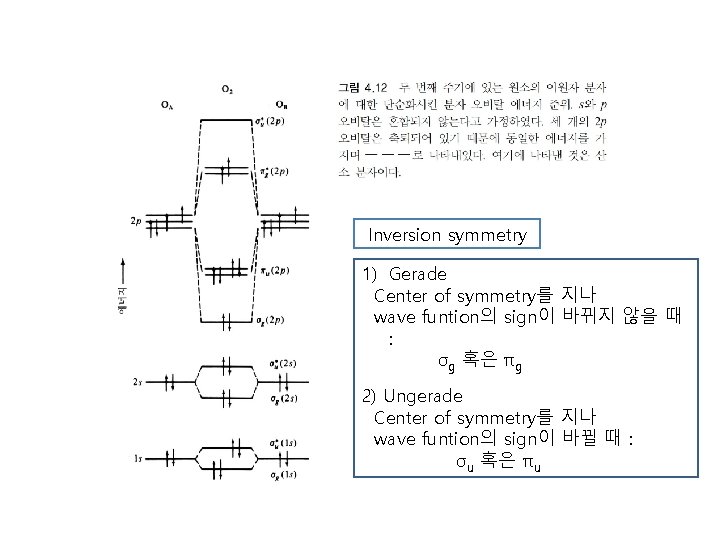
Inversion symmetry 1) Gerade Center of symmetry를 지나 wave funtion의 sign이 바뀌지 않을 때 : σg 혹은 πg 2) Ungerade Center of symmetry를 지나 wave funtion의 sign이 바뀔 때 : σu 혹은 πu
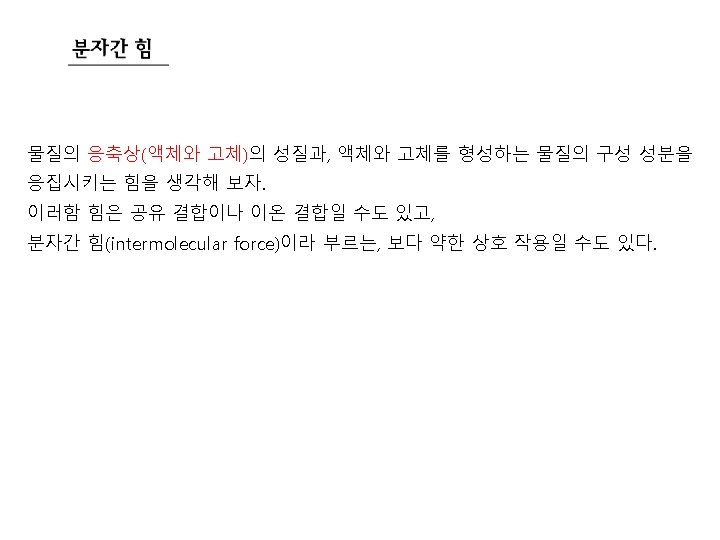
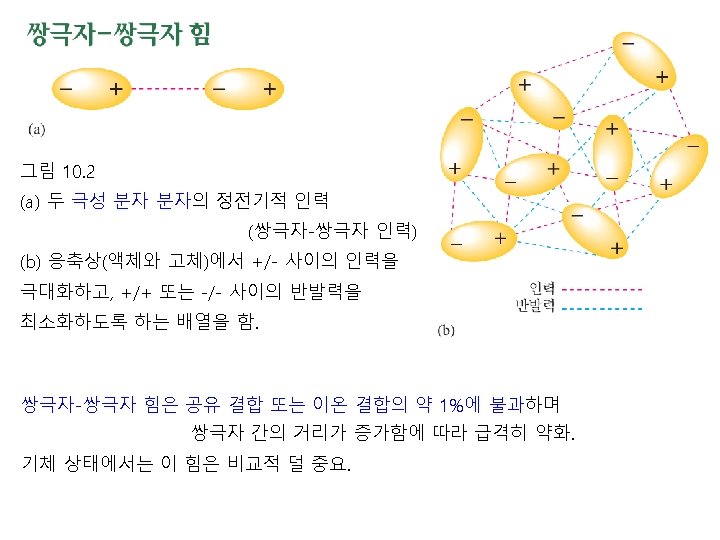
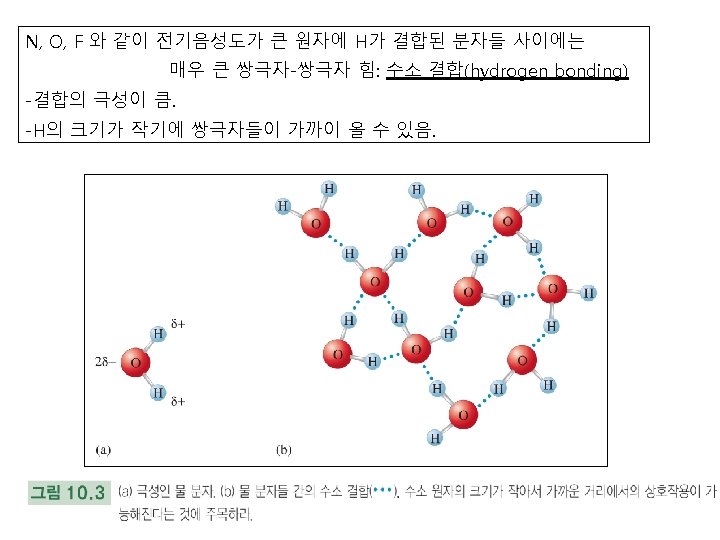
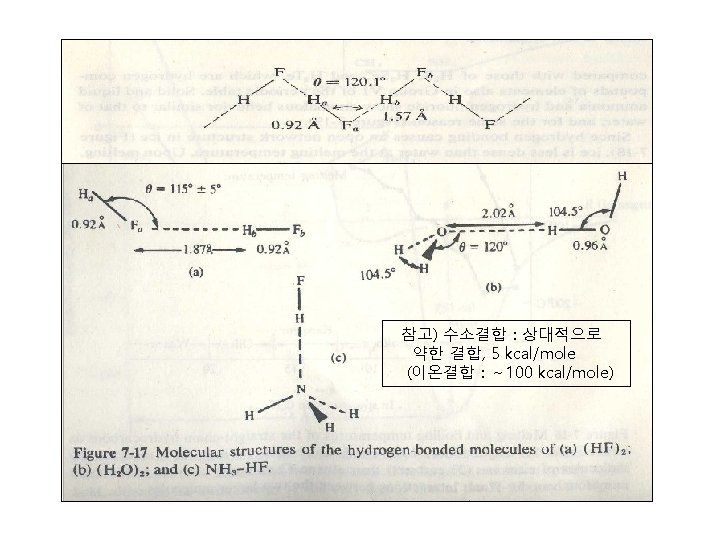
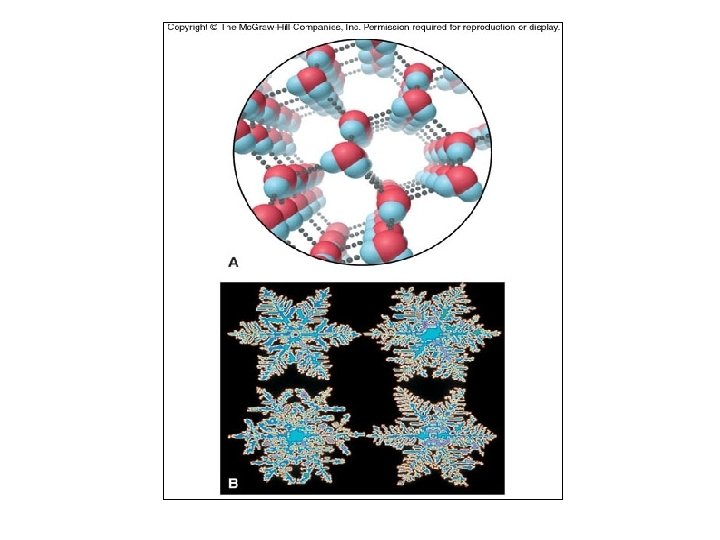
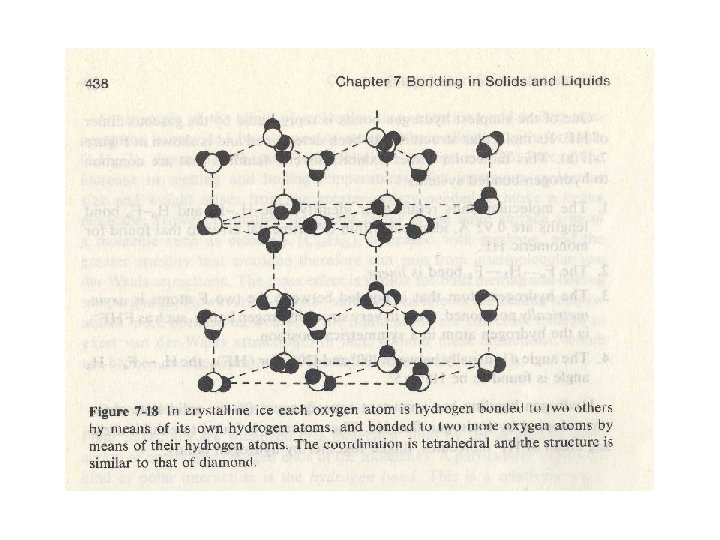
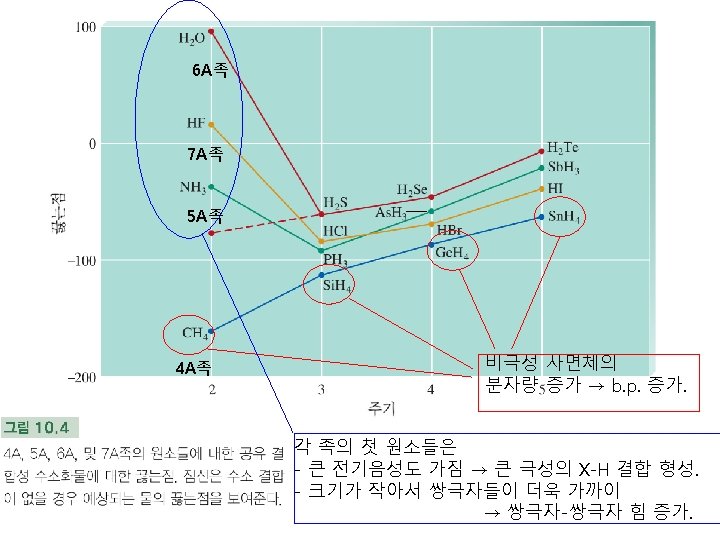
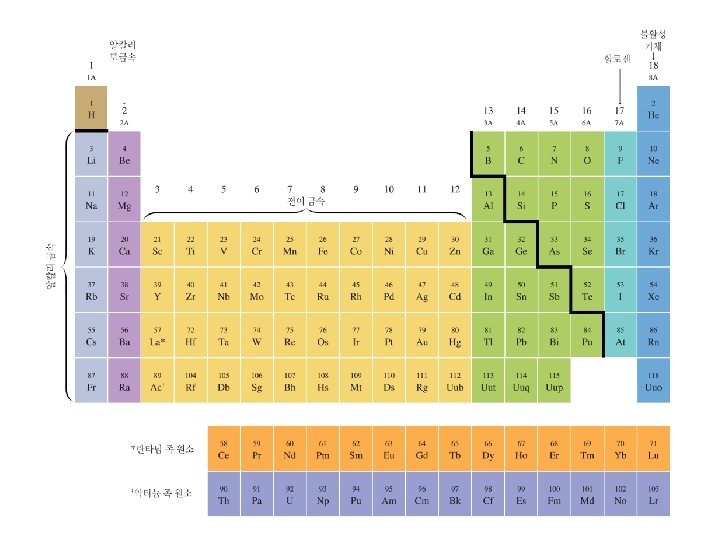
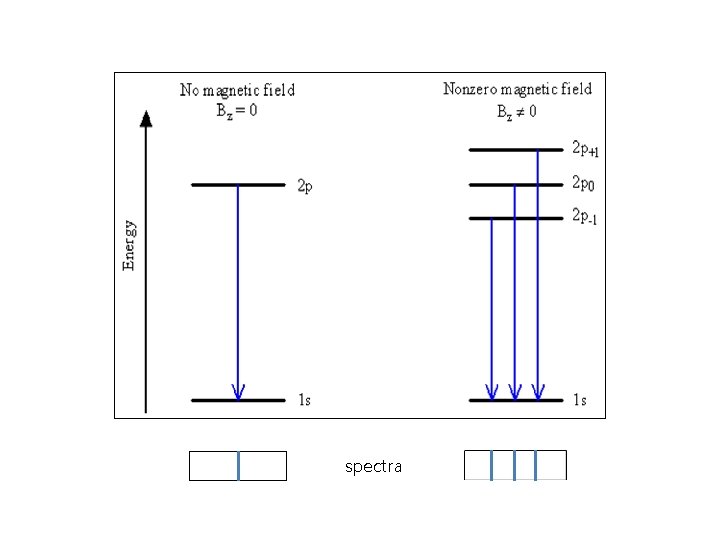
spectra
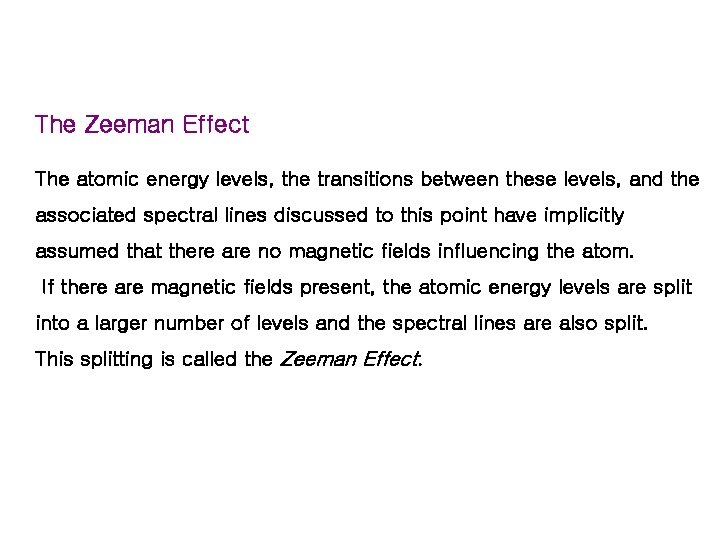
The Zeeman Effect The atomic energy levels, the transitions between these levels, and the associated spectral lines discussed to this point have implicitly assumed that there are no magnetic fields influencing the atom. If there are magnetic fields present, the atomic energy levels are split into a larger number of levels and the spectral lines are also split. This splitting is called the Zeeman Effect.
Khan academy jj thomson
Perkembangan model atom
Picasso 1897
The structure of the atom section 2 defining the atom
Relative atomic mass of beryllium
Periodic table trends
Periodic table ionic size
Atomic mass of oxygen
Atomic
Atomic number vs atomic radius
Atoms family atomic math challenge doc
Lesson 11 atomic pudding models of the atom
Atomic alchemy
Atomic structure and properties ap chemistry
Basic atomic structure worksheet
Democritus diagram
Atomic structure of conductors
First ionization energy definition ib
Ib chemistry atomic structure
Atomic theory graphic organizer
Atomic theory timeline project
Z atomic symbol
Tungsten atomic structure
Nuclear symbol notation
Atoms poster
Atomic structure graphic organizer
Timeline of atomic structure
Ap chemistry atomic structure and periodicity
Unit 4 atomic structure
Atomic history webquest
Diamond structure apf
Piezoelectric crystal atomic structure
Boardworks atomic structure
Atomic structure of semiconductor
Chapter 4 atomic structure vocabulary
Atomic structure
Nonmetals are located where on the periodic table
Atomic structure
Ape man practice
Oxygen periodic trends
Bohr rutherford diagram magnesium
Photoelectric absorption
What does the atomic number represent
Inter atomic bonding
Discovery of atomic structure
Atomic structure
Bohr radius of hydrogen atom
Atomicity of elements
Atomic structure
How many neutrons does francium have
Democritus and aristotle
Chapter 7 atomic structure and periodicity
Hát kết hợp bộ gõ cơ thể
Bổ thể
Tỉ lệ cơ thể trẻ em
Voi kéo gỗ như thế nào
Tư thế worms-breton
Chúa yêu trần thế alleluia
Các môn thể thao bắt đầu bằng từ đua
Thế nào là hệ số cao nhất
Các châu lục và đại dương trên thế giới
Công thức tính độ biến thiên đông lượng
Trời xanh đây là của chúng ta thể thơ
Mật thư anh em như thể tay chân
Làm thế nào để 102-1=99
Phản ứng thế ankan
Các châu lục và đại dương trên thế giới
Thơ thất ngôn tứ tuyệt đường luật
Quá trình desamine hóa có thể tạo ra
Một số thể thơ truyền thống
Cái miệng nó xinh thế
Vẽ hình chiếu vuông góc của vật thể sau
Nguyên nhân của sự mỏi cơ sinh 8
đặc điểm cơ thể của người tối cổ
Thế nào là giọng cùng tên? *
Vẽ hình chiếu đứng bằng cạnh của vật thể
Fecboak
Thẻ vin