Relative Humidity Supersaturation Formation of Cloud Droplets Prof
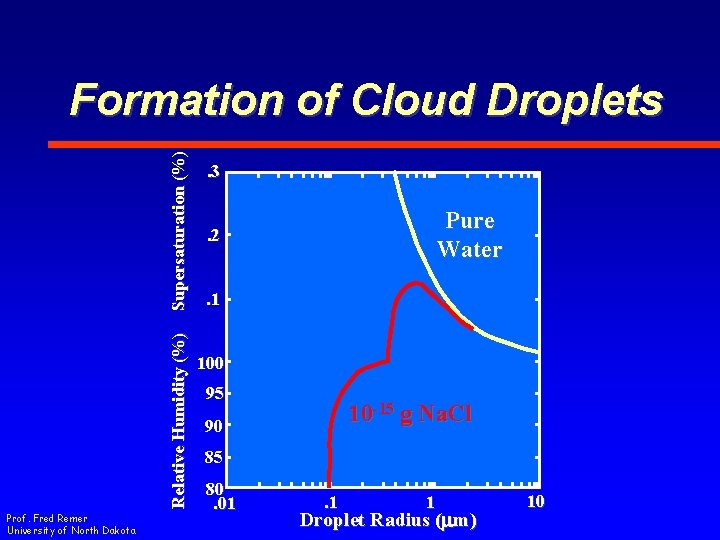
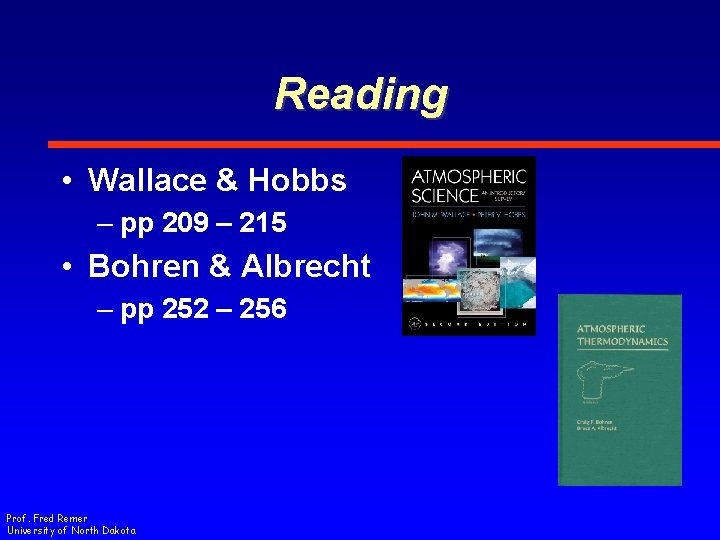
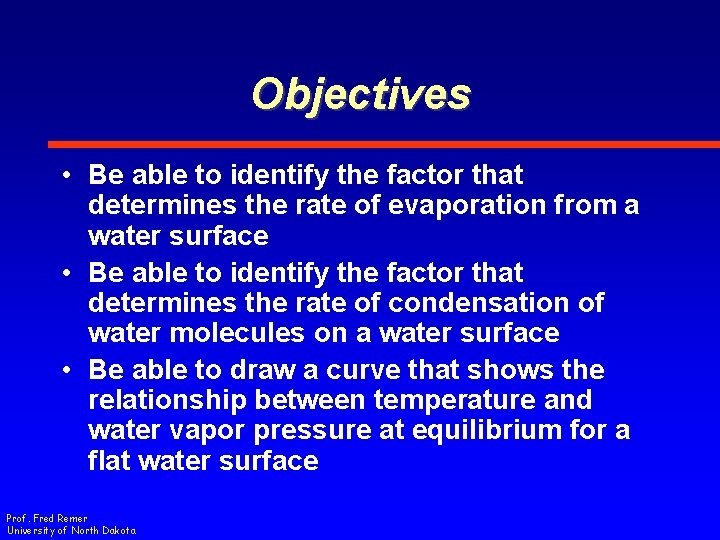
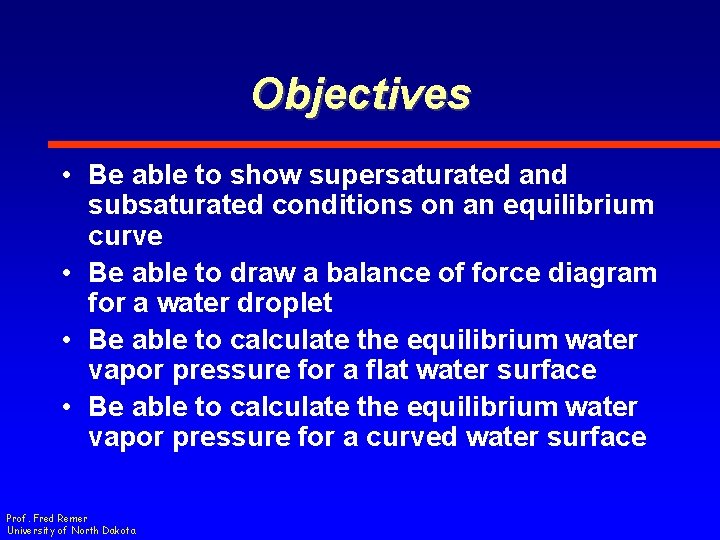
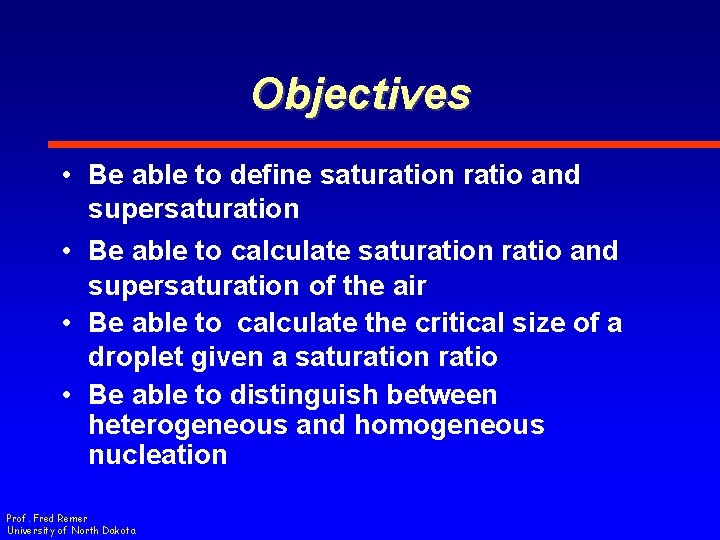
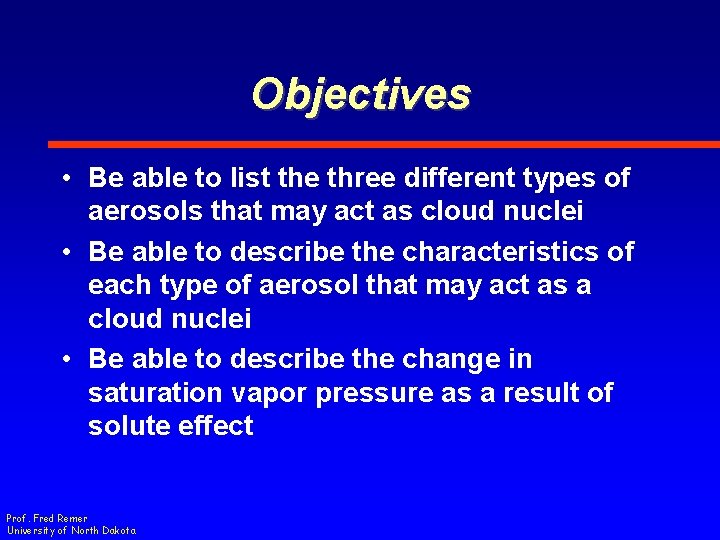
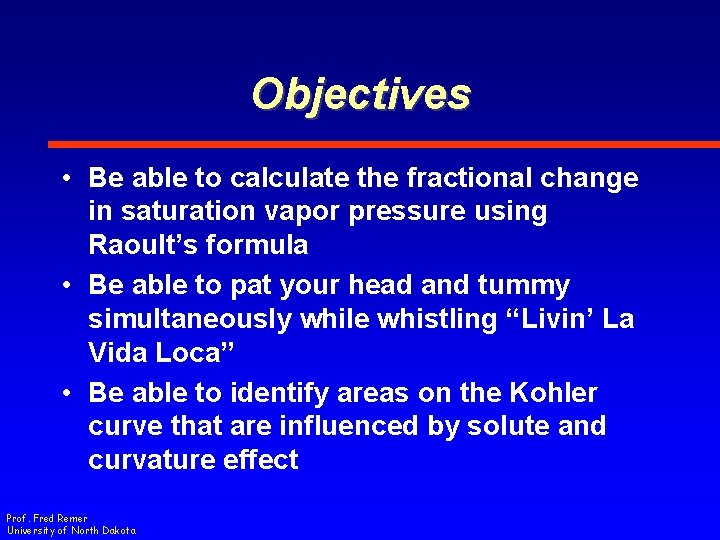
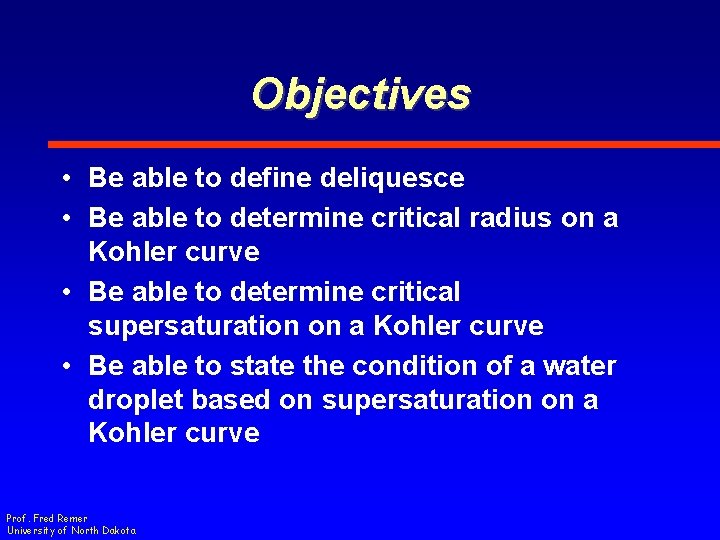
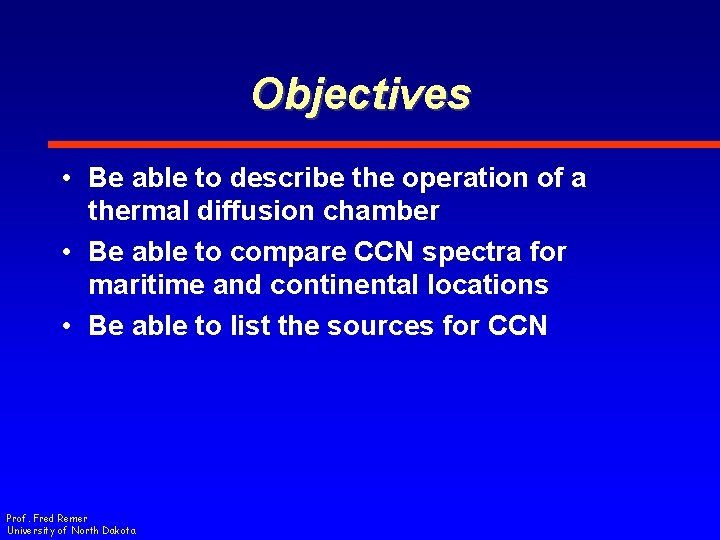
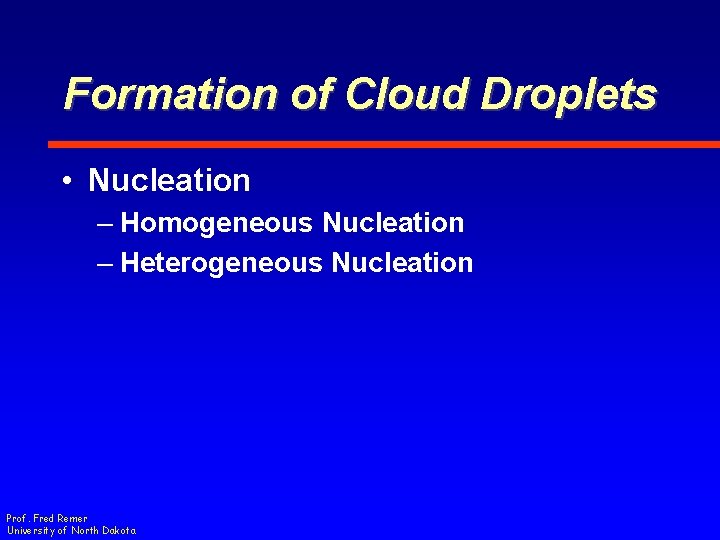
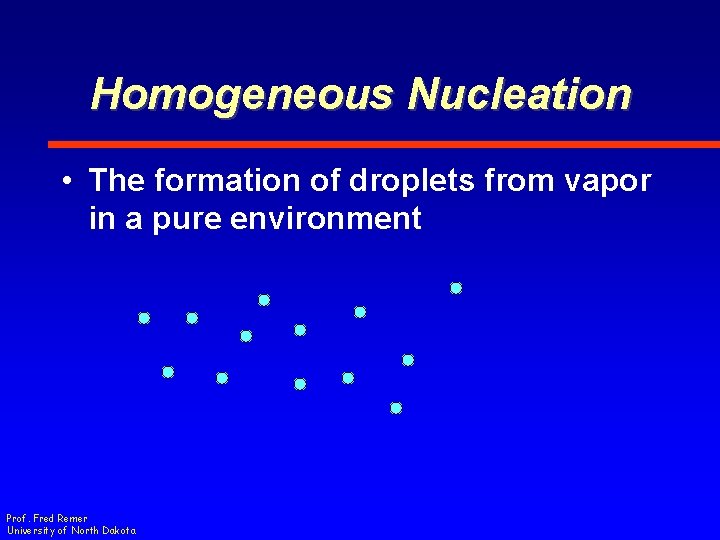
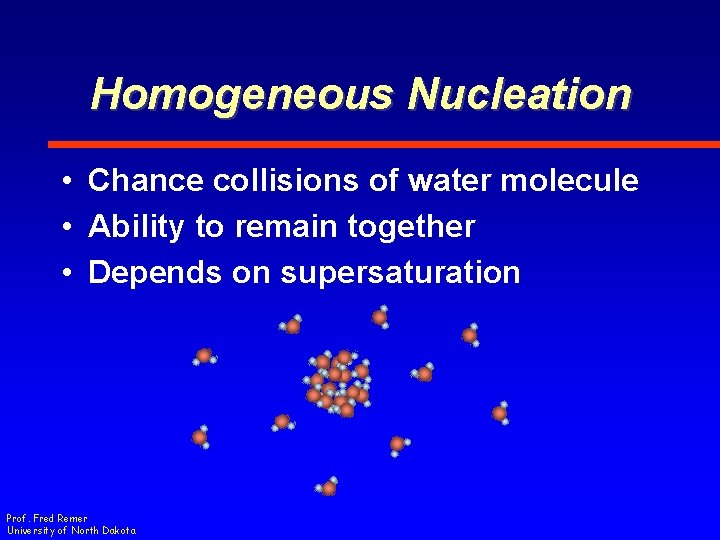
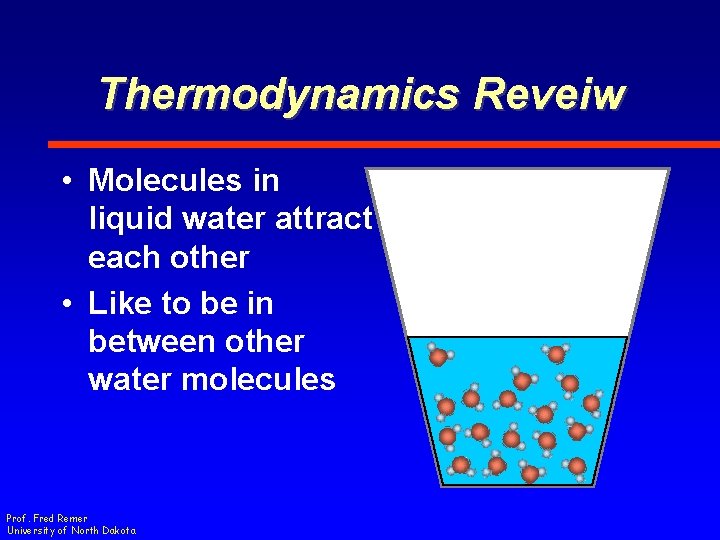
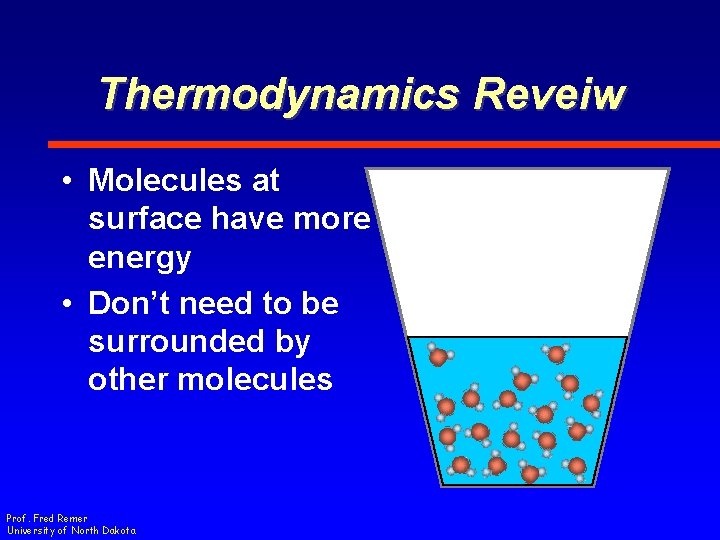
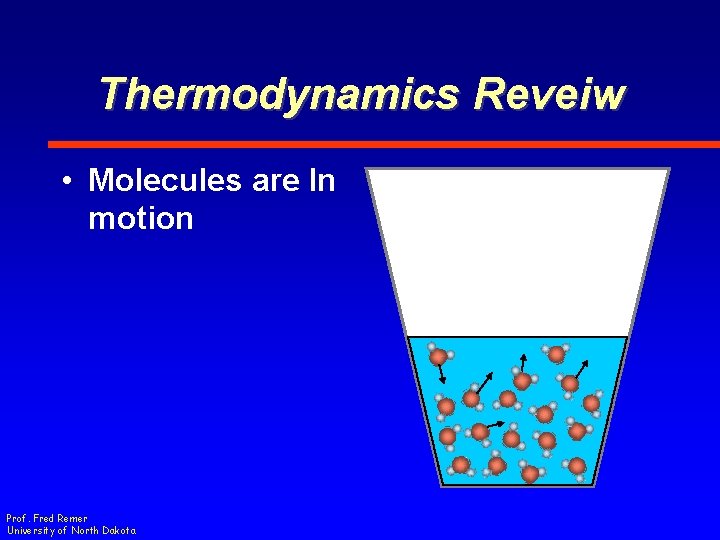
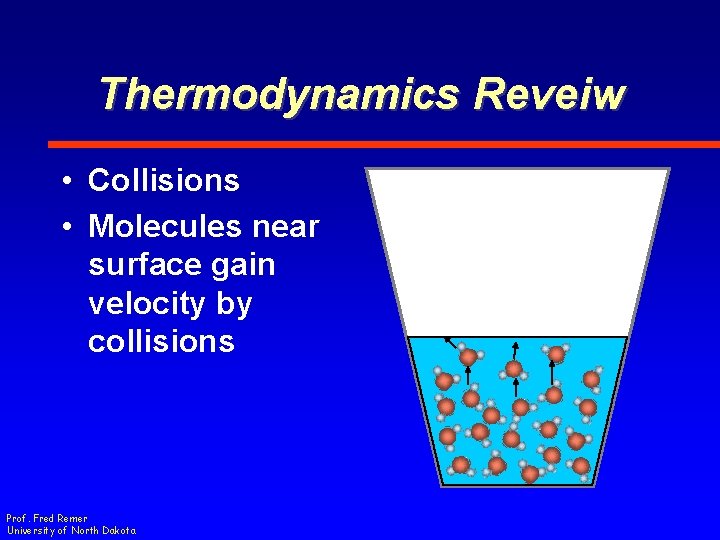
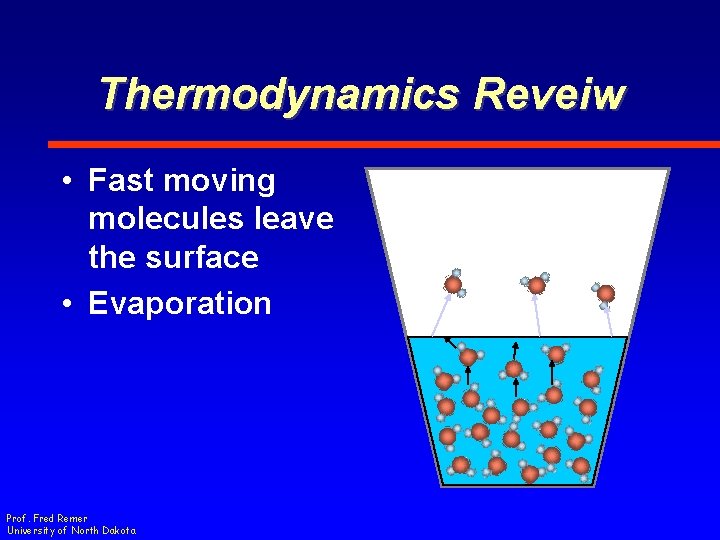
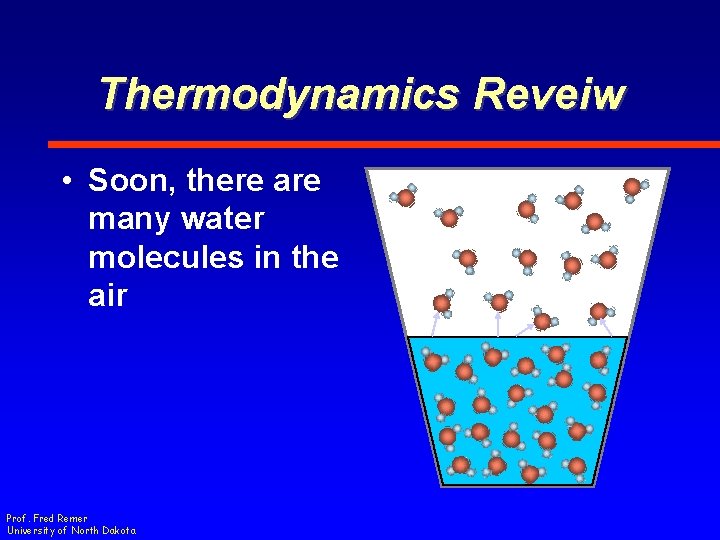
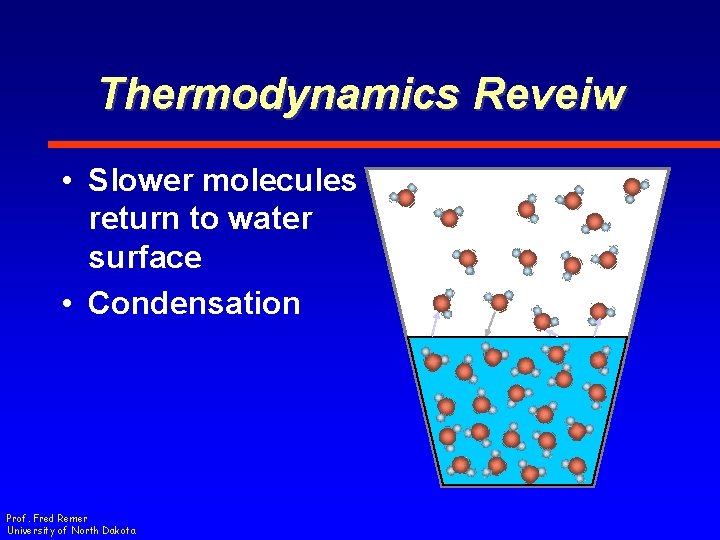
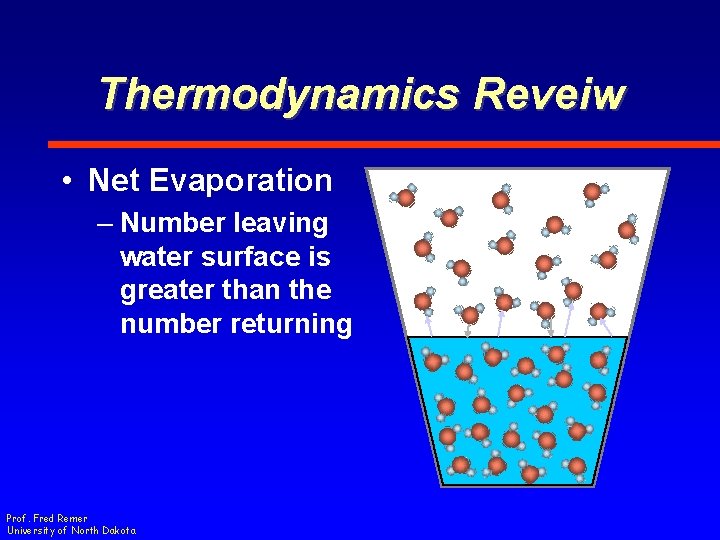
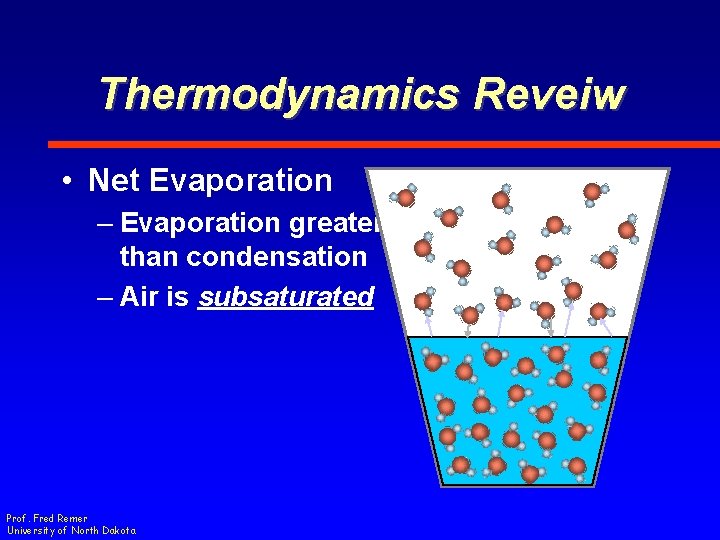
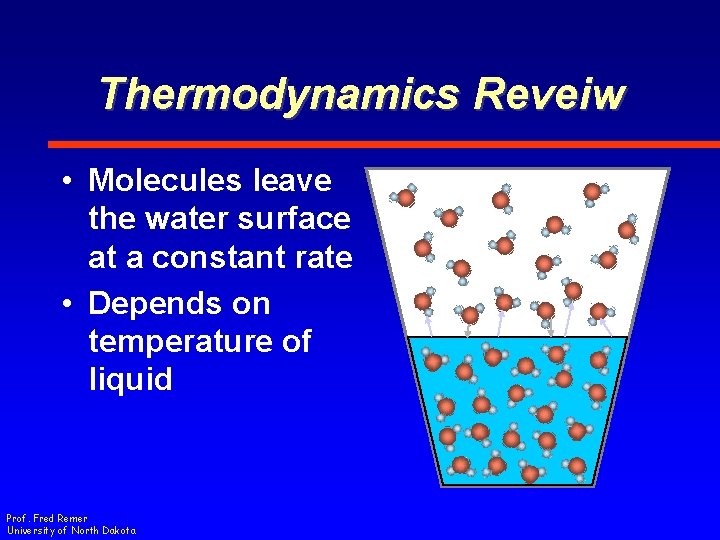
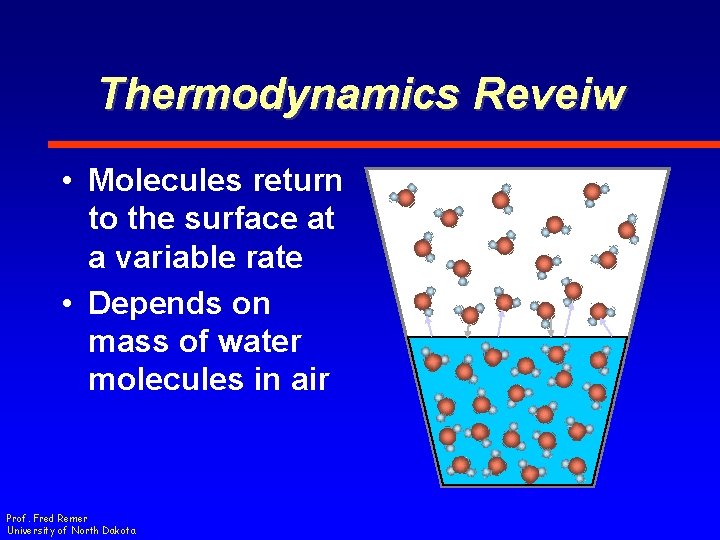
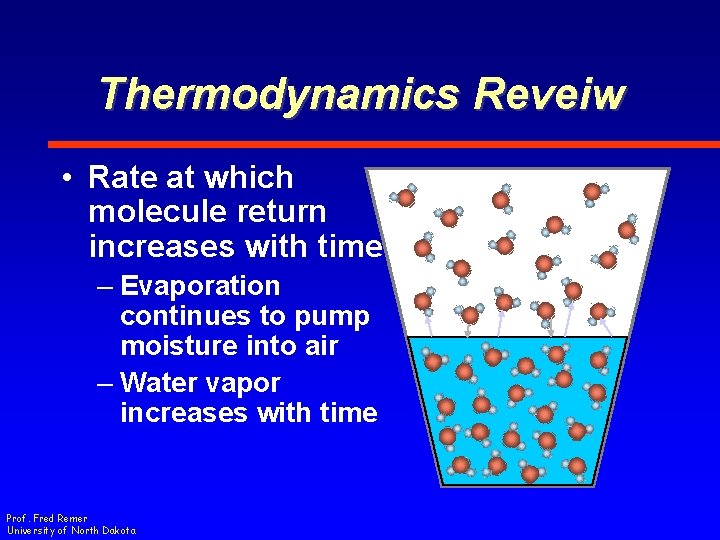
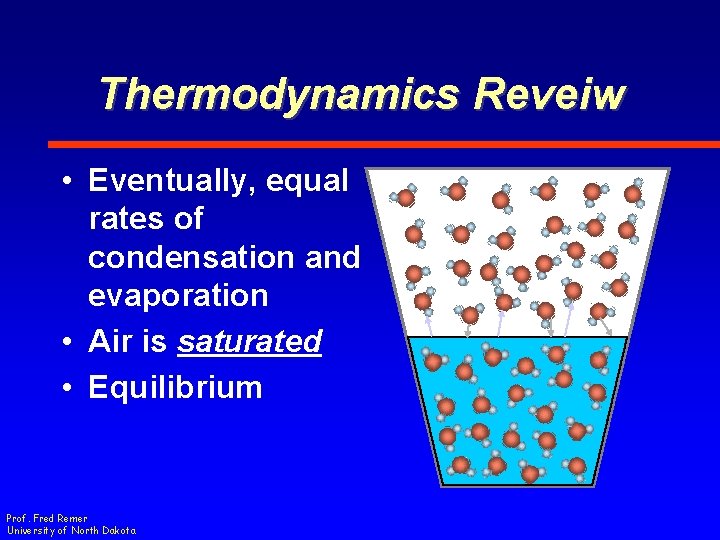
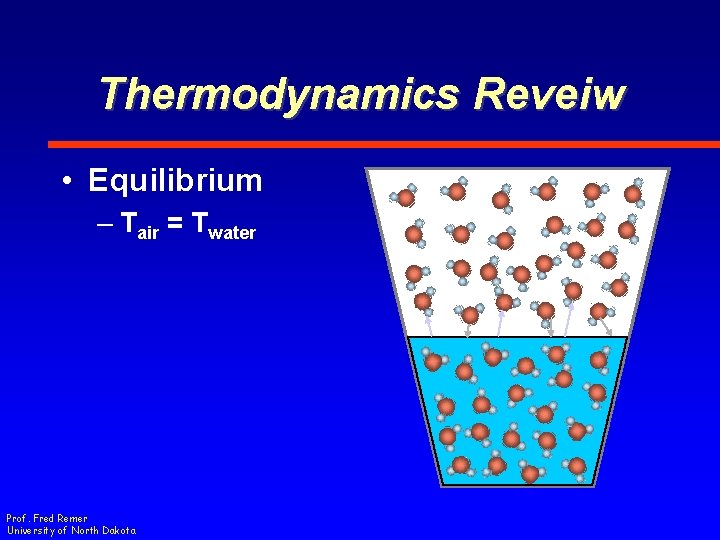
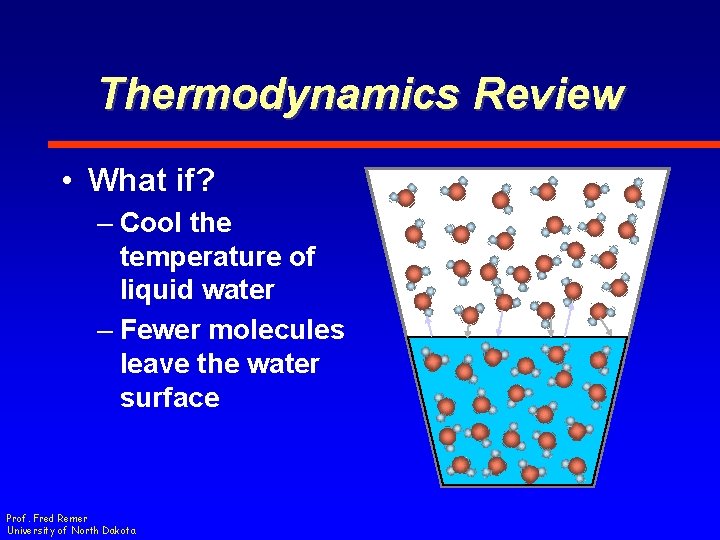
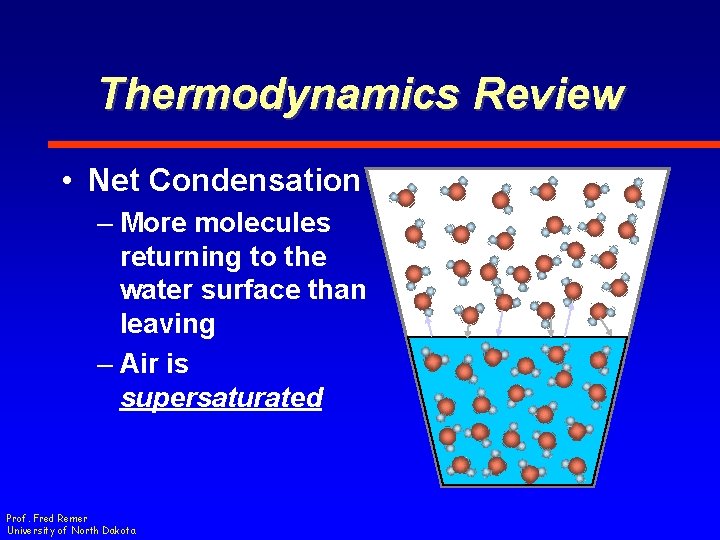
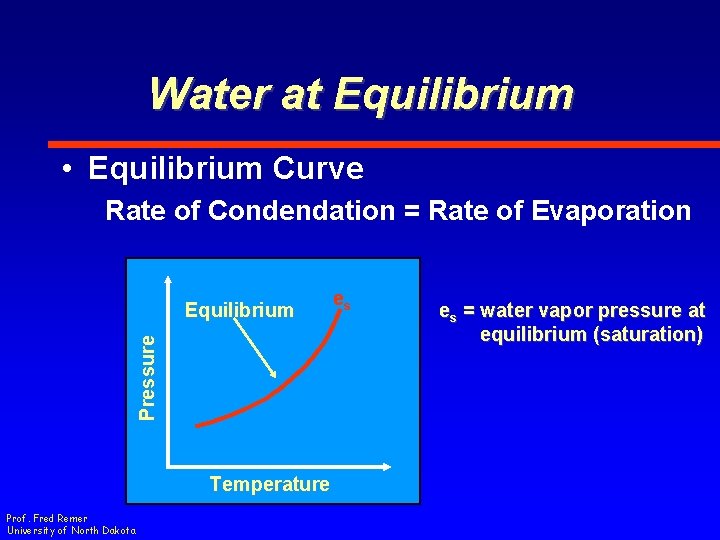
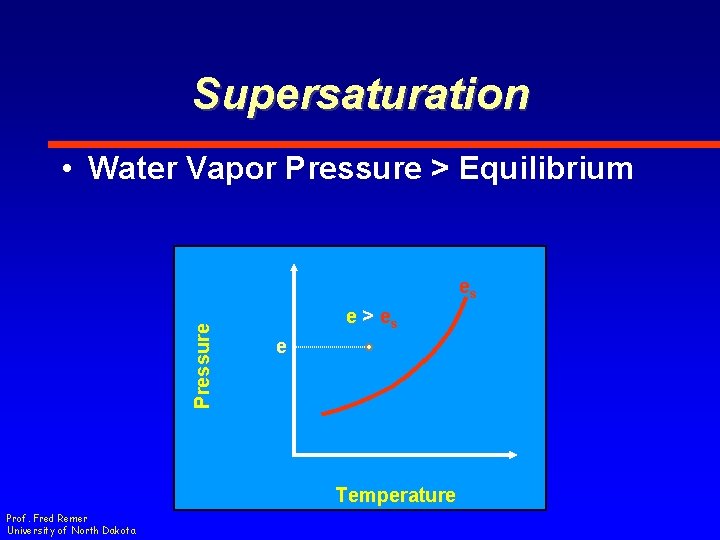
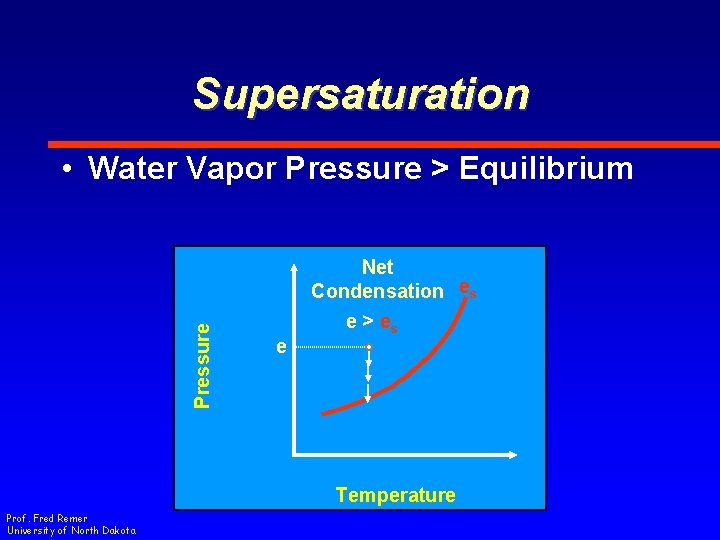
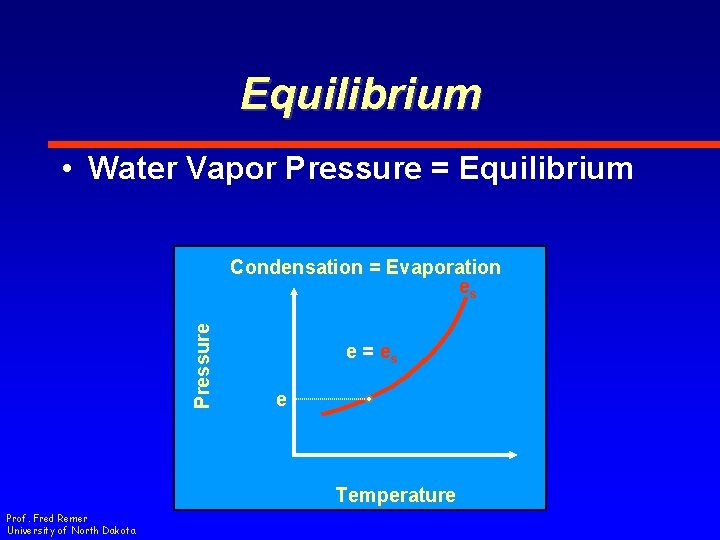
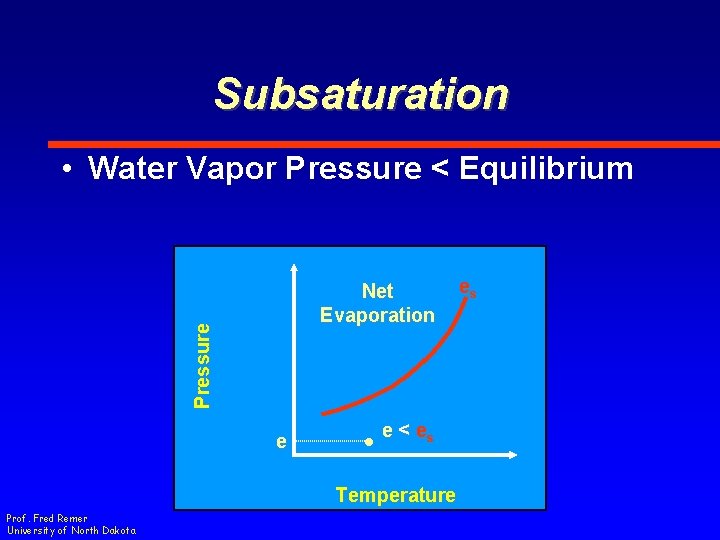
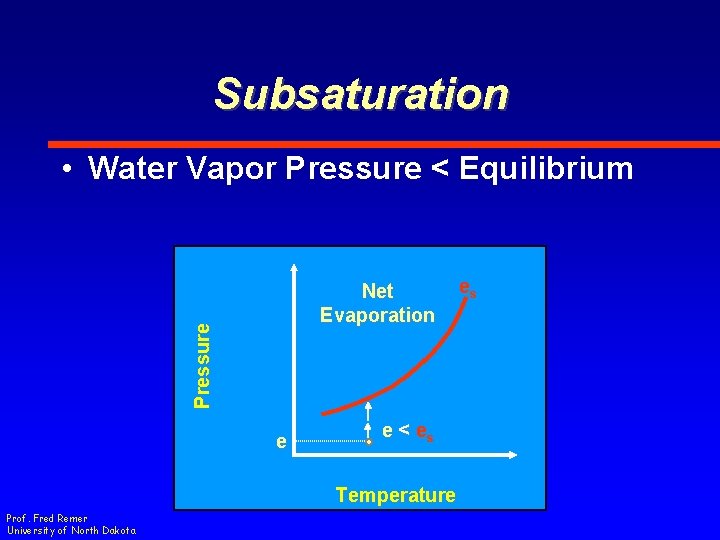
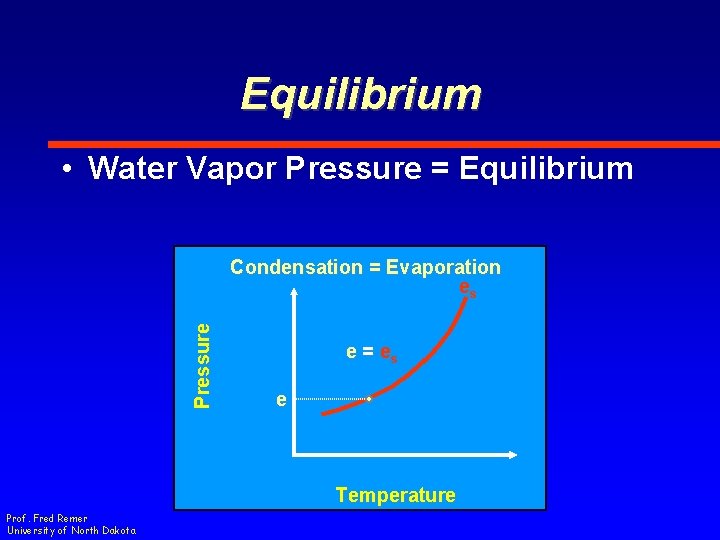
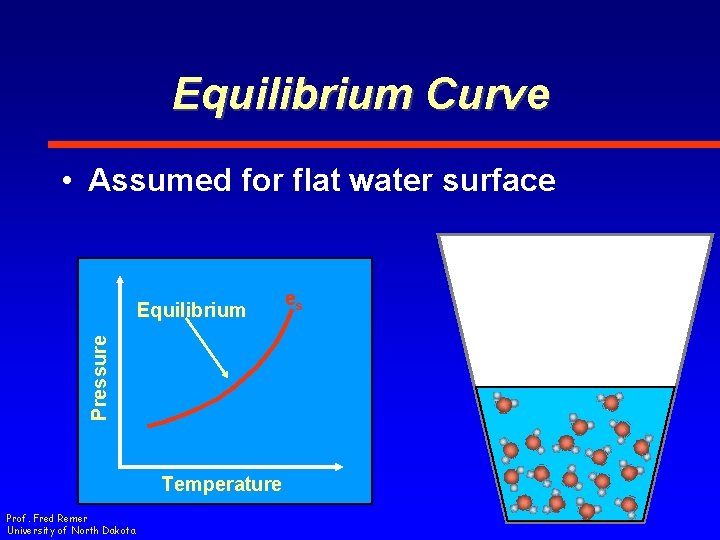
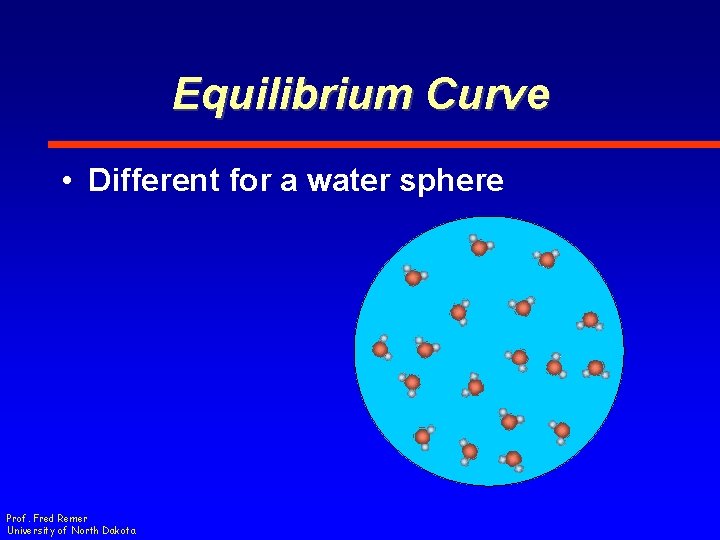
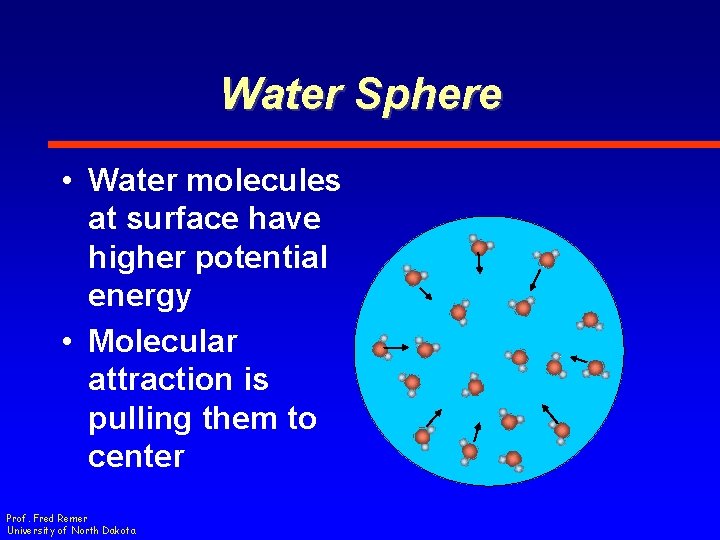
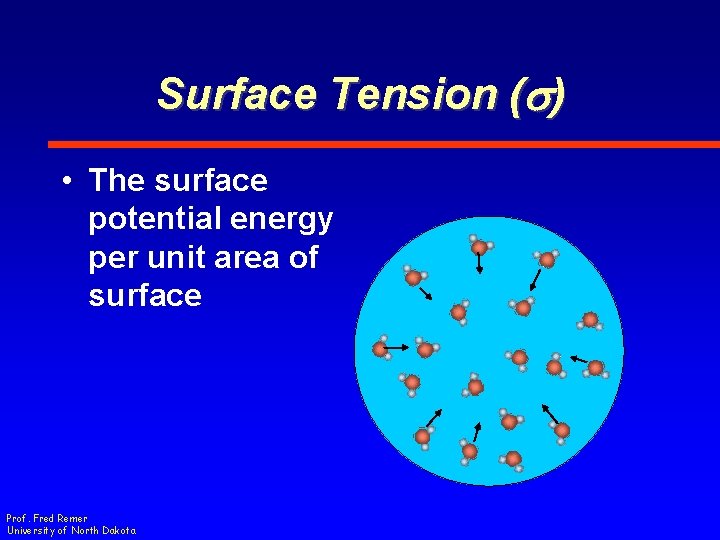
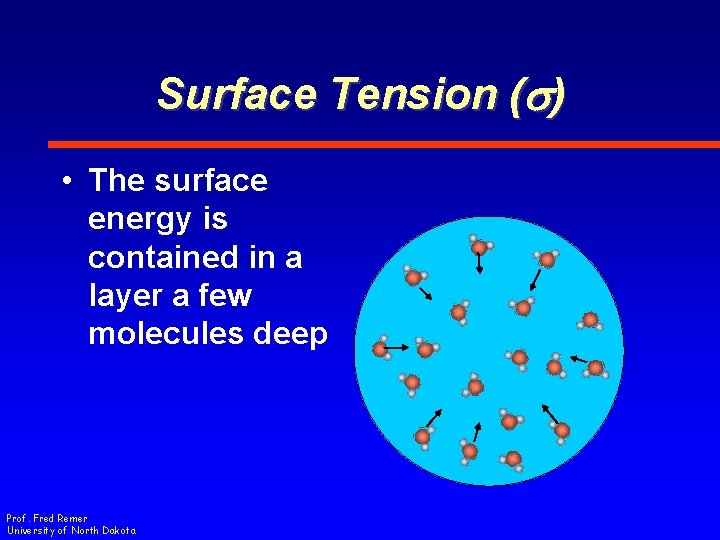
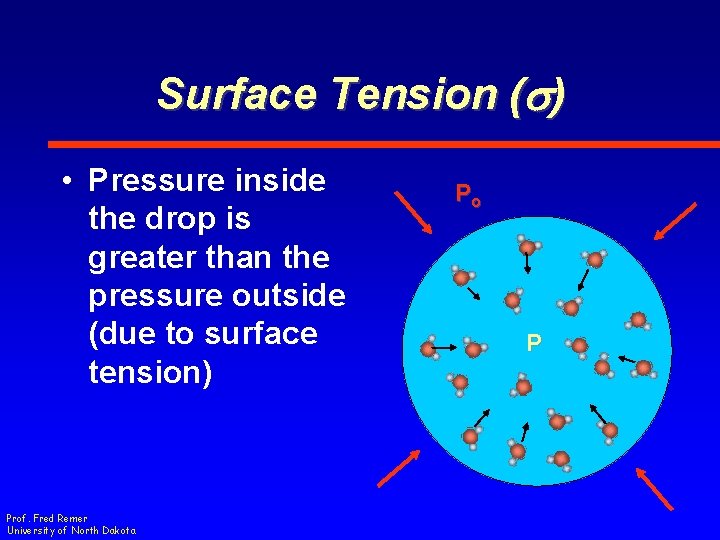
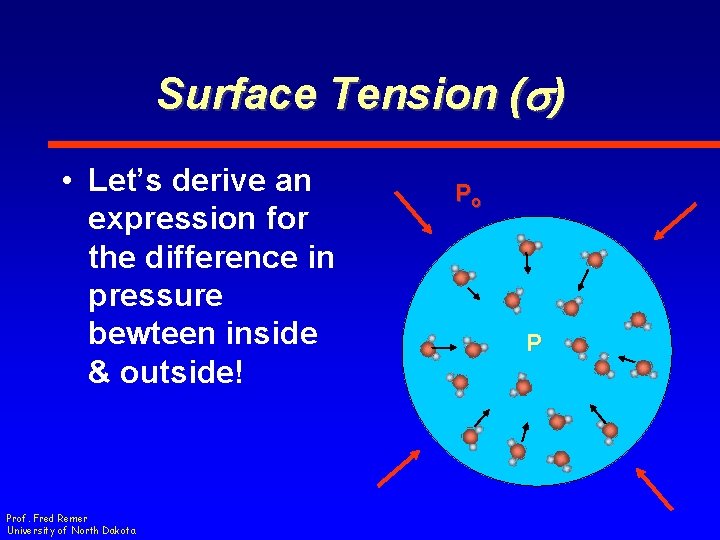
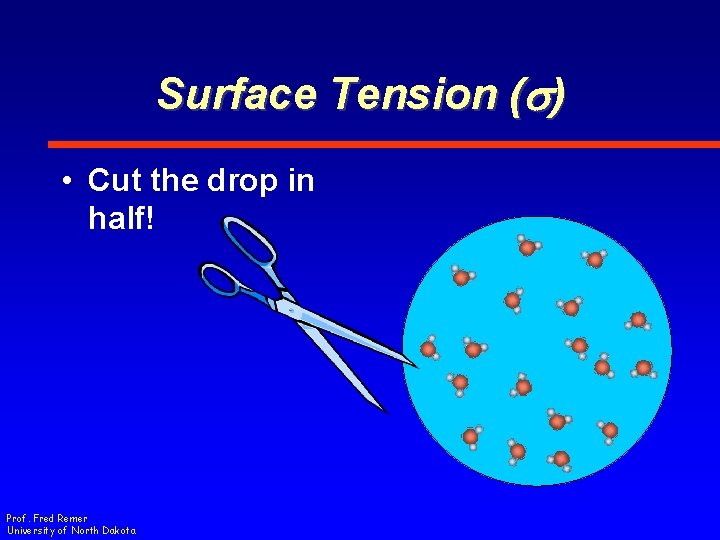
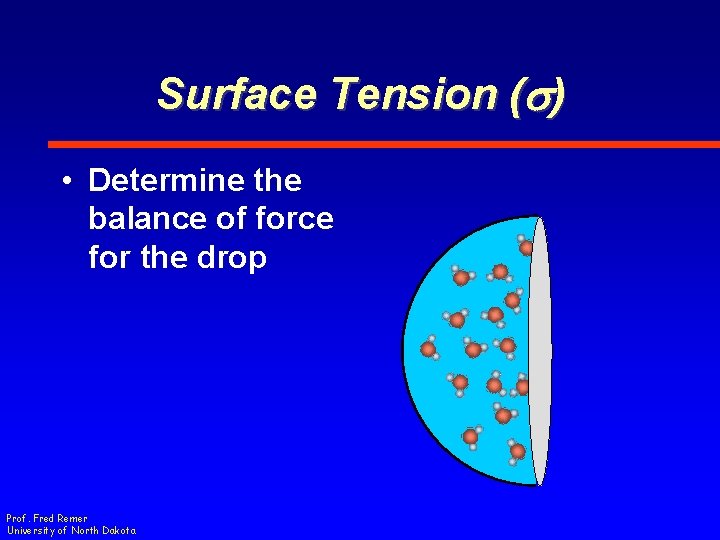
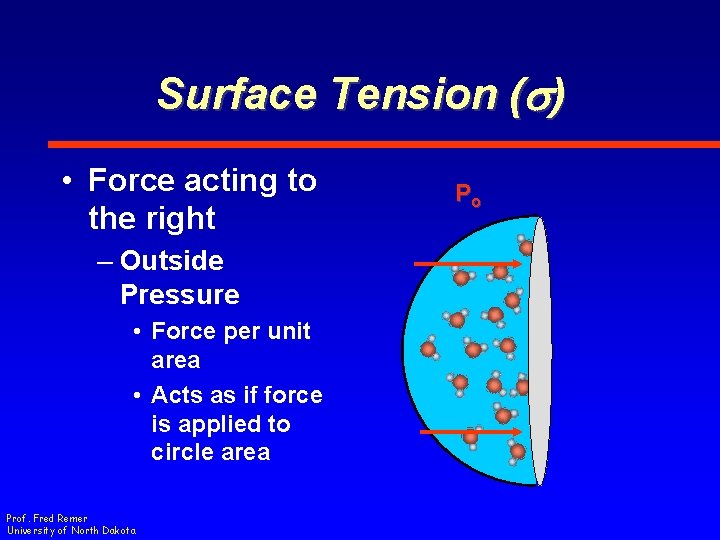
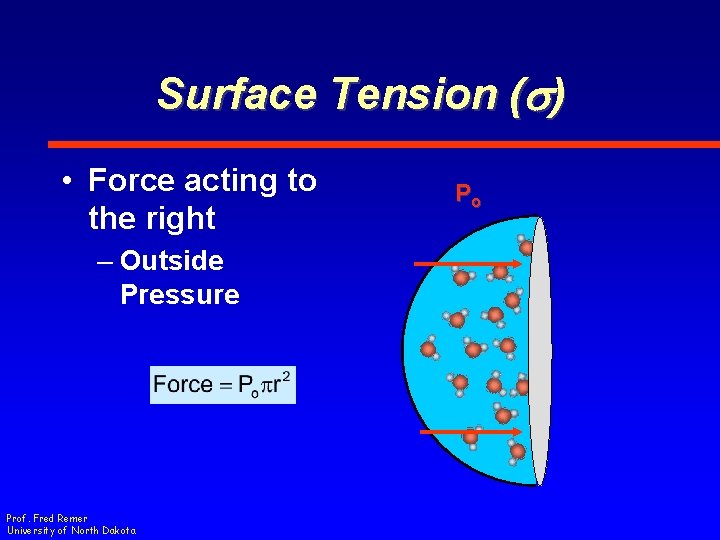
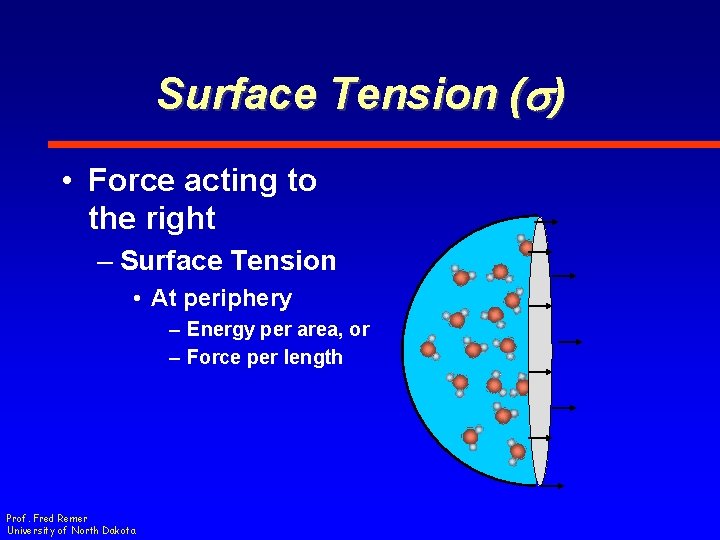
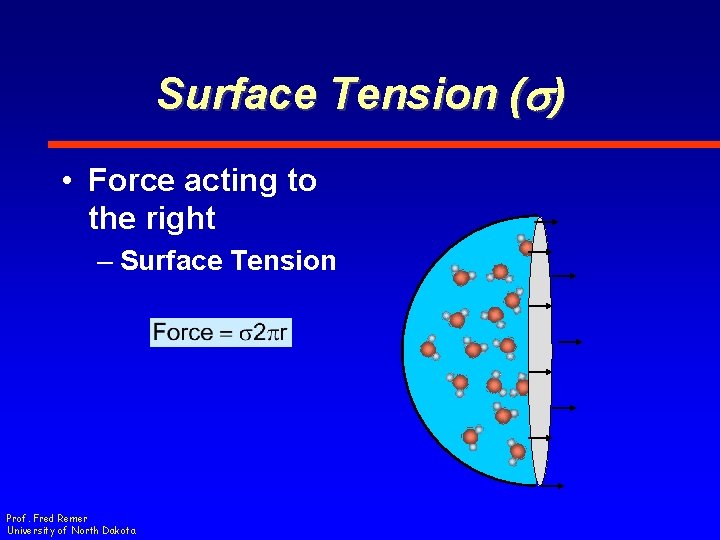
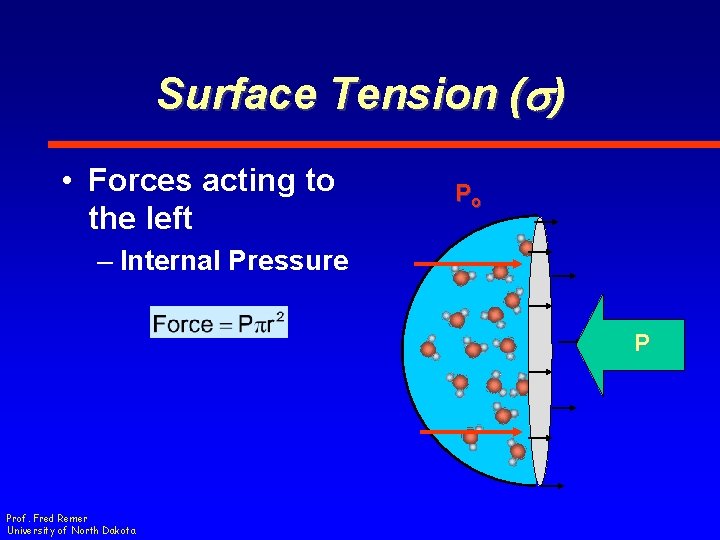
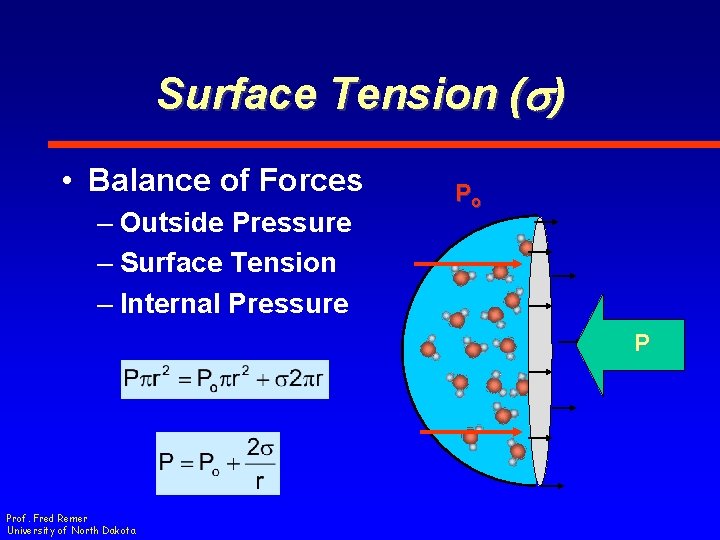
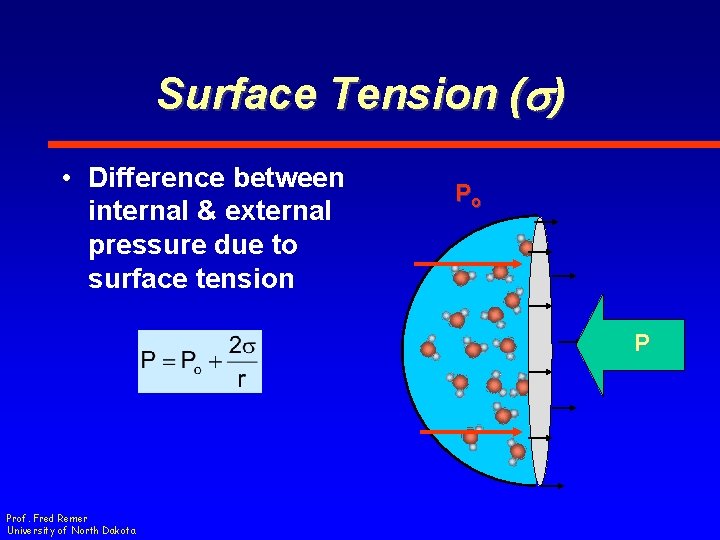
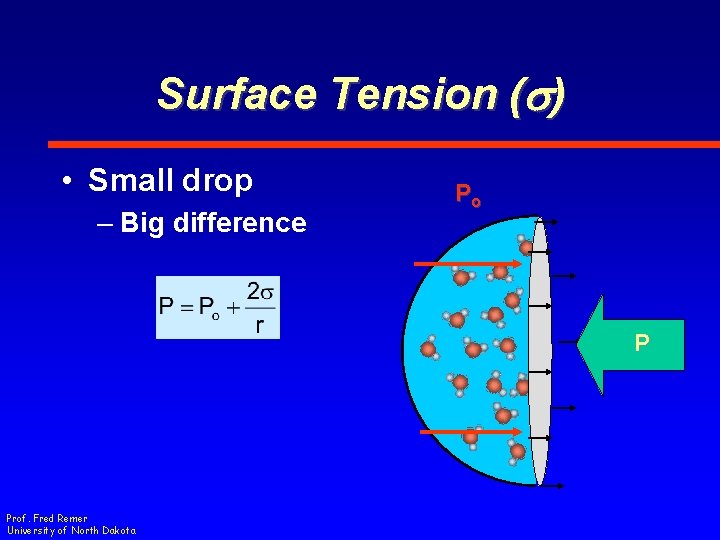
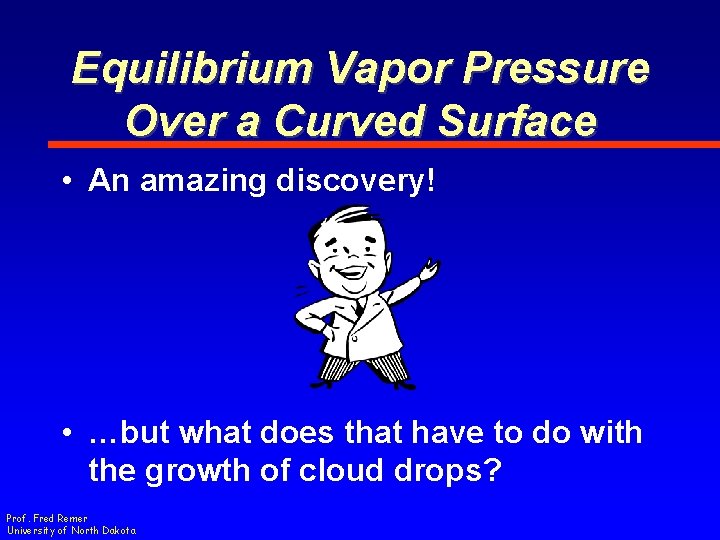
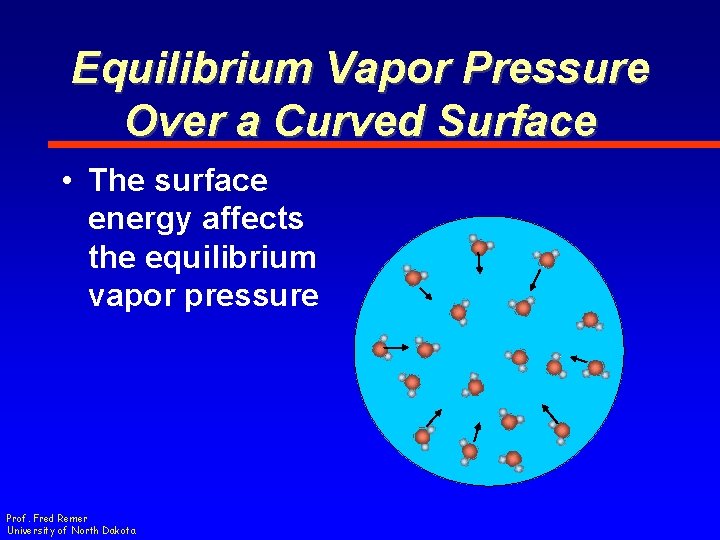
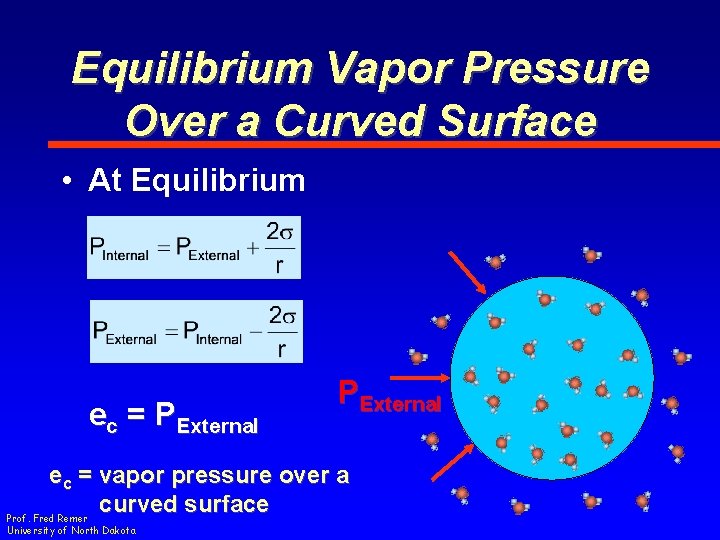
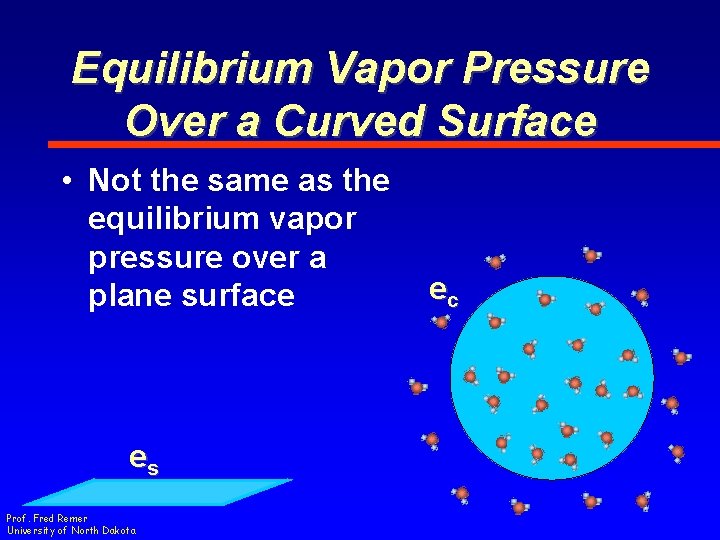
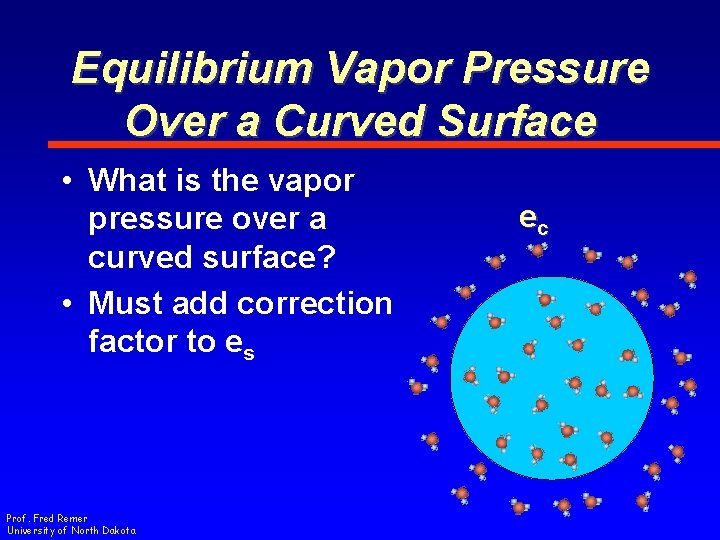
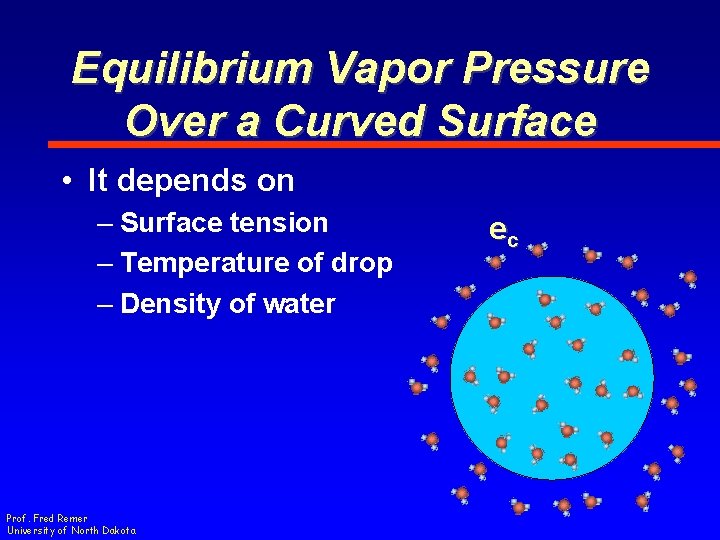
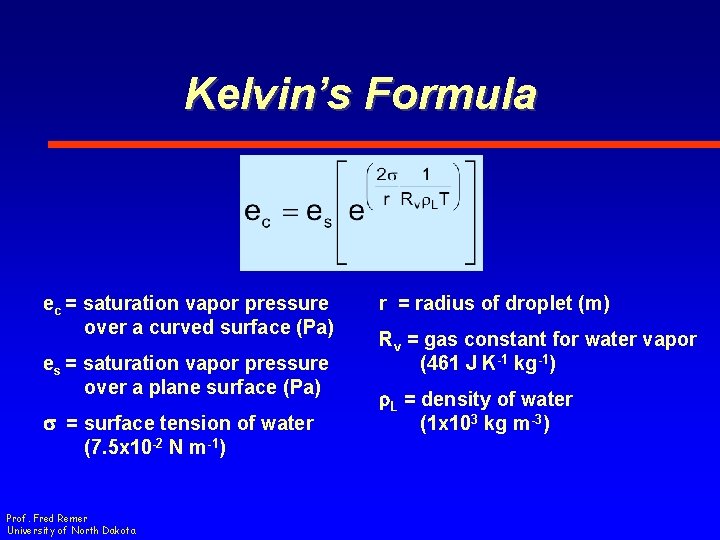
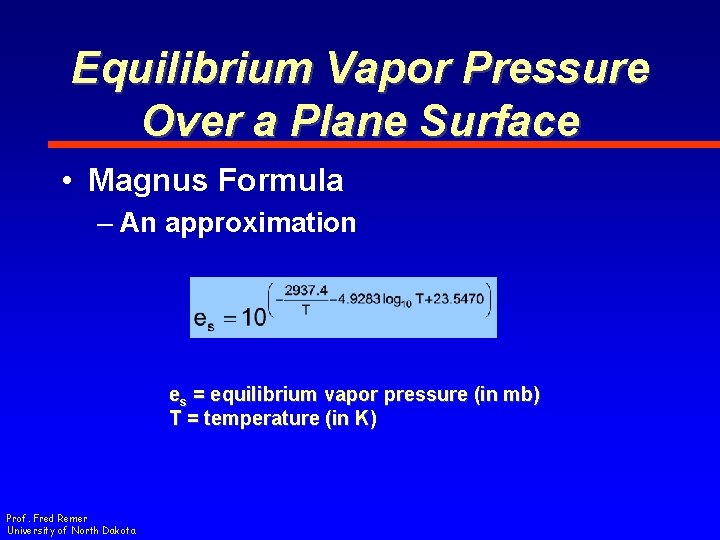
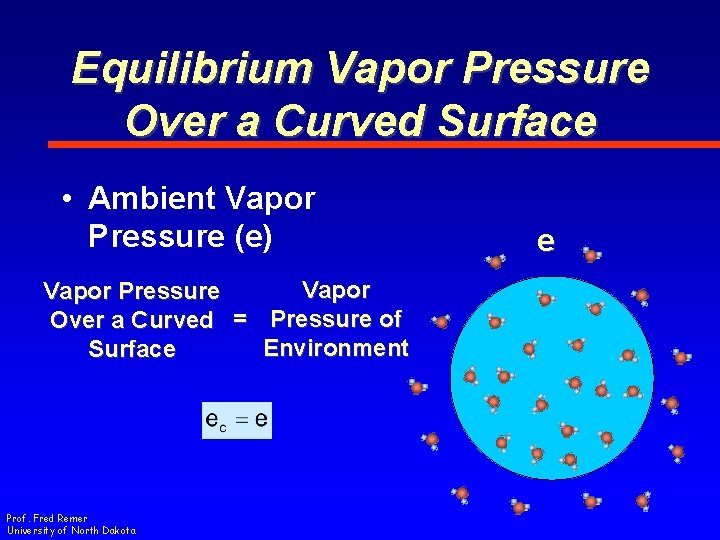
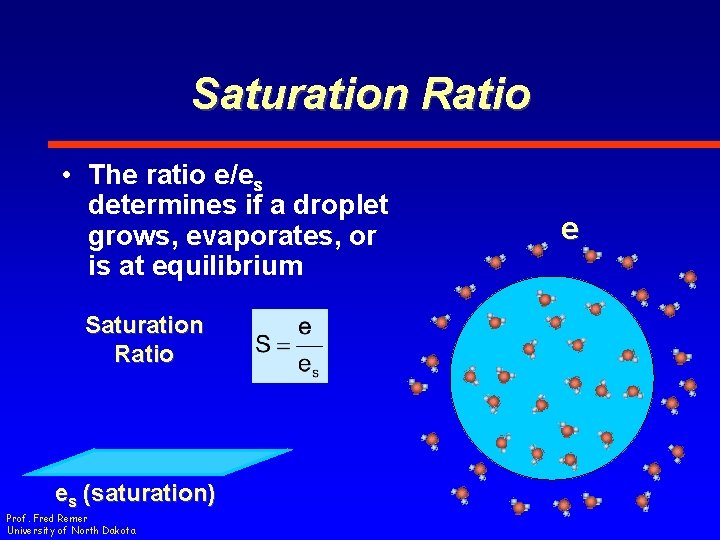
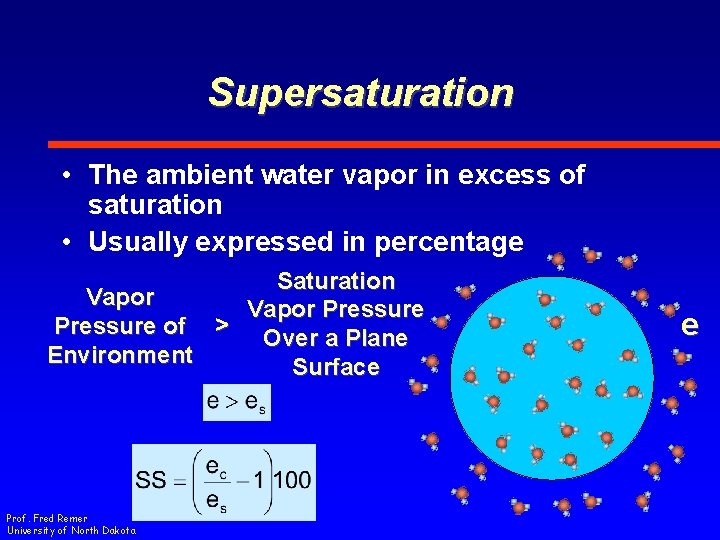
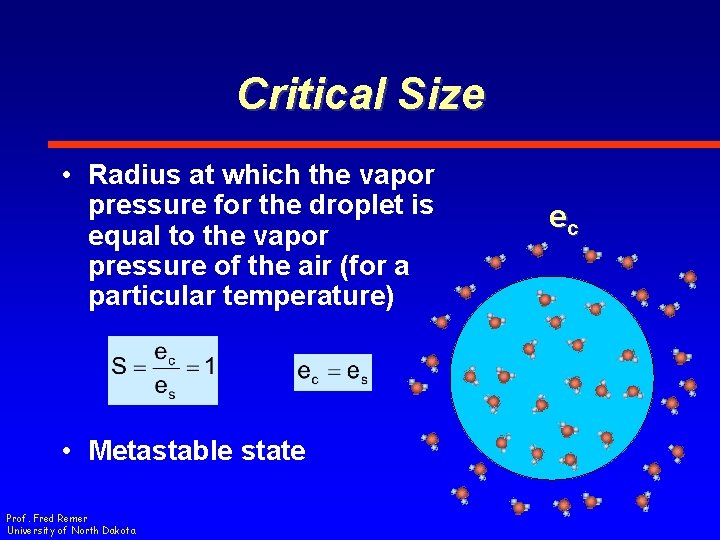
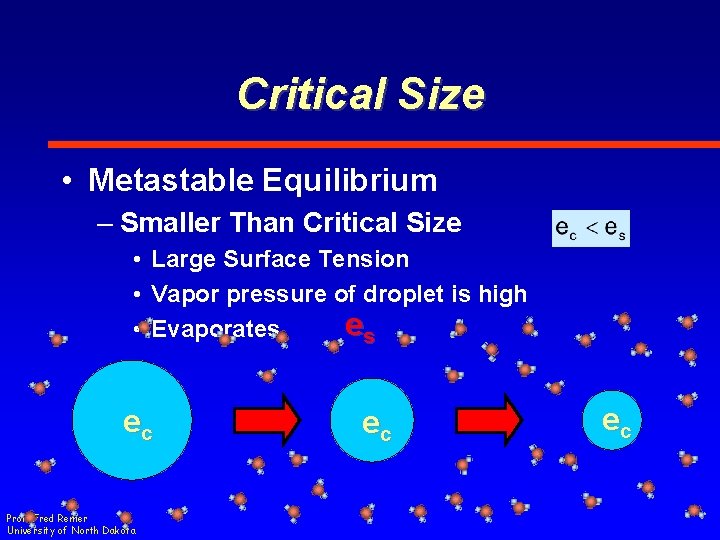
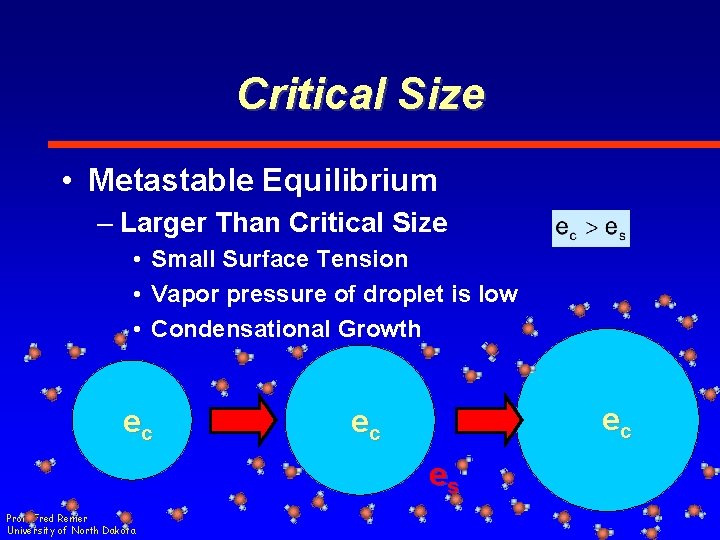
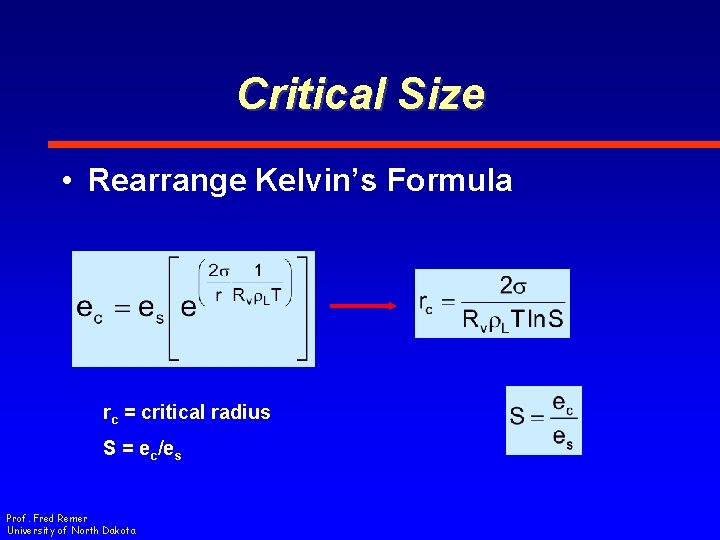
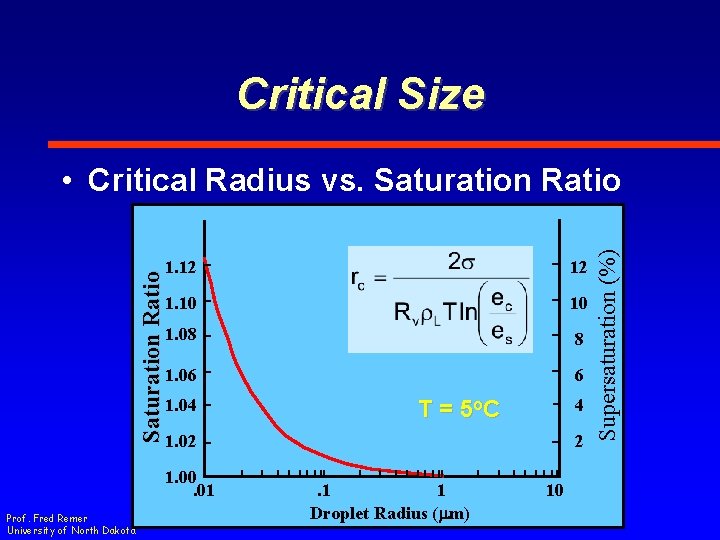
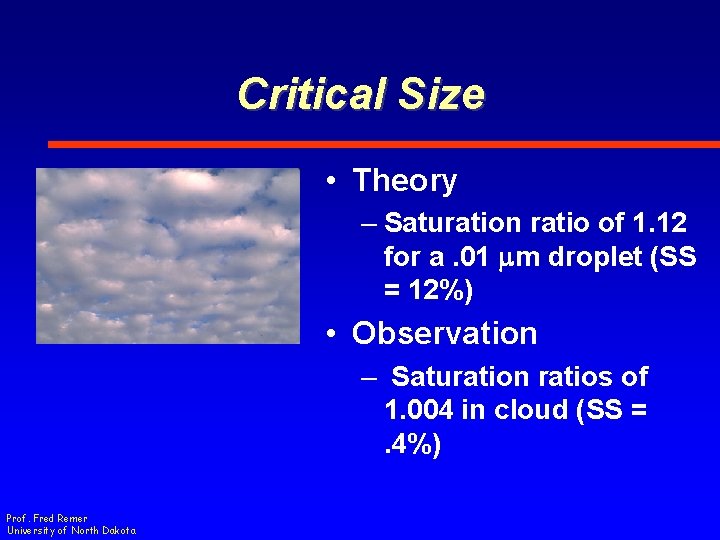
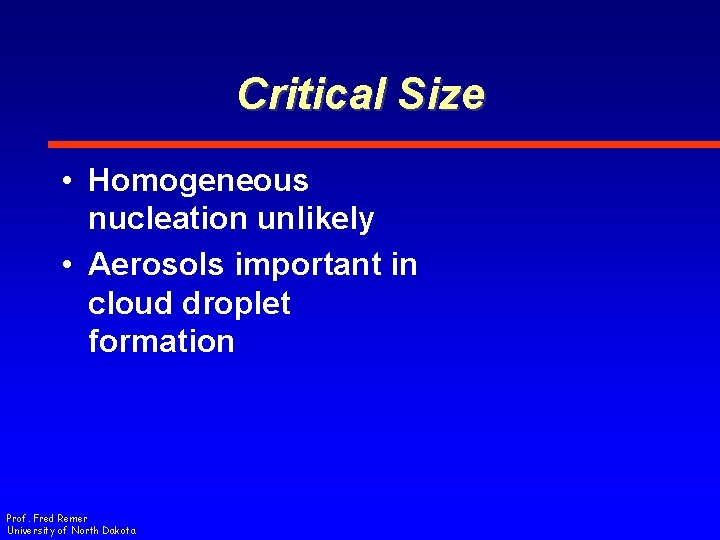
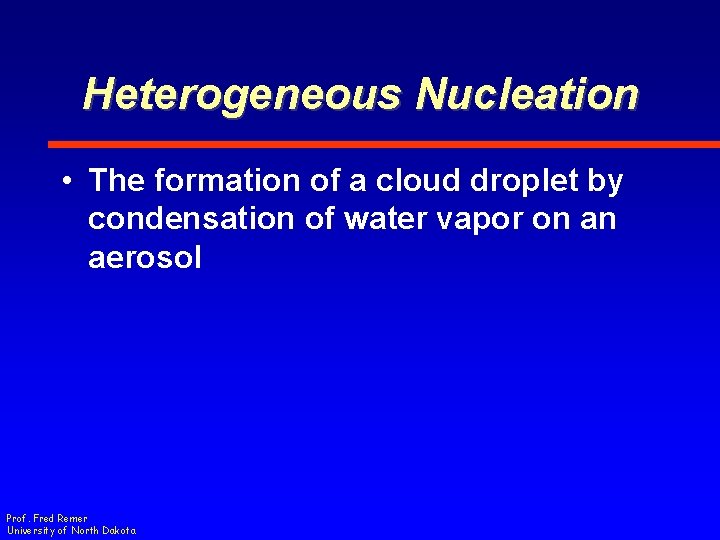
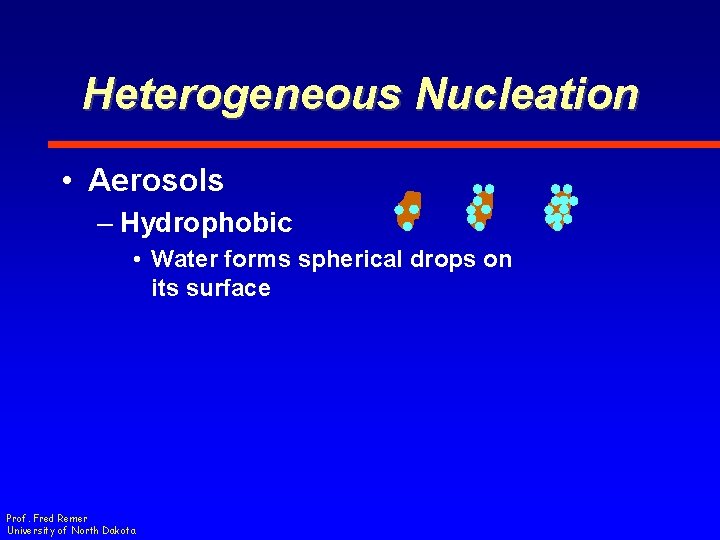
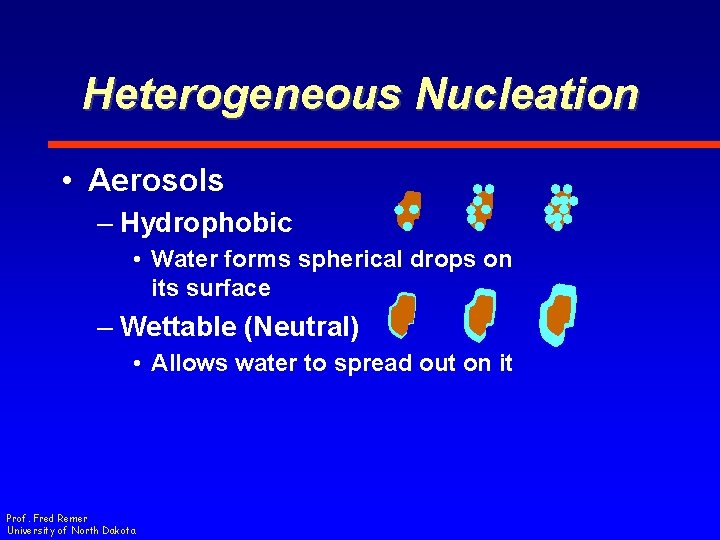
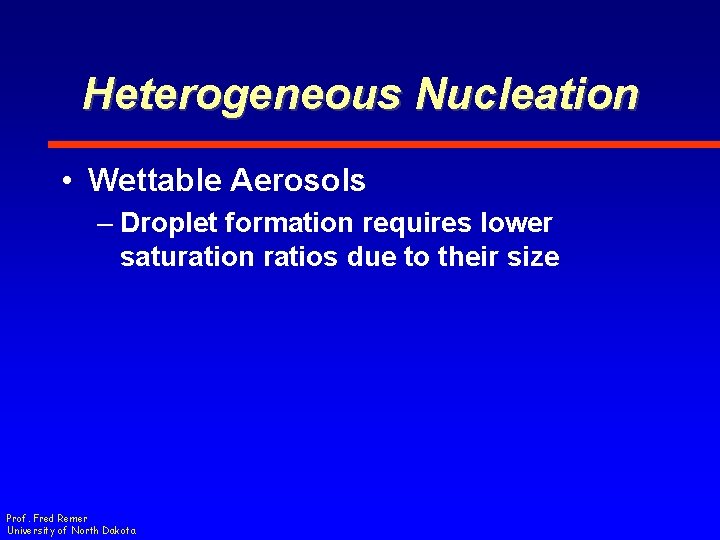
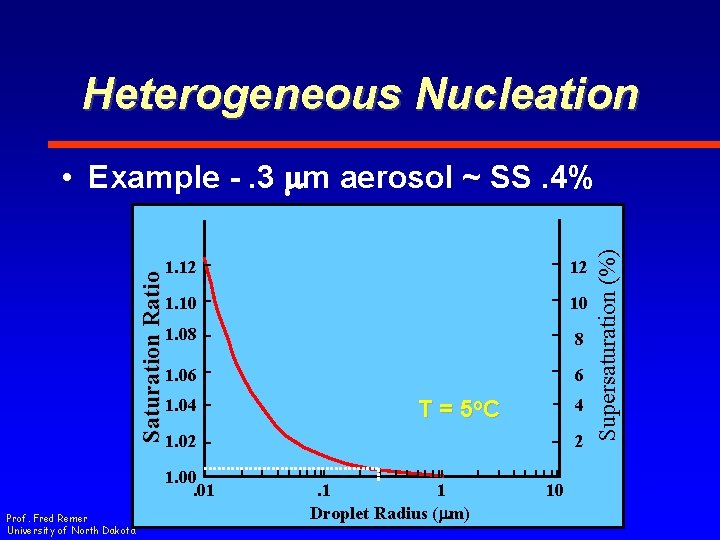
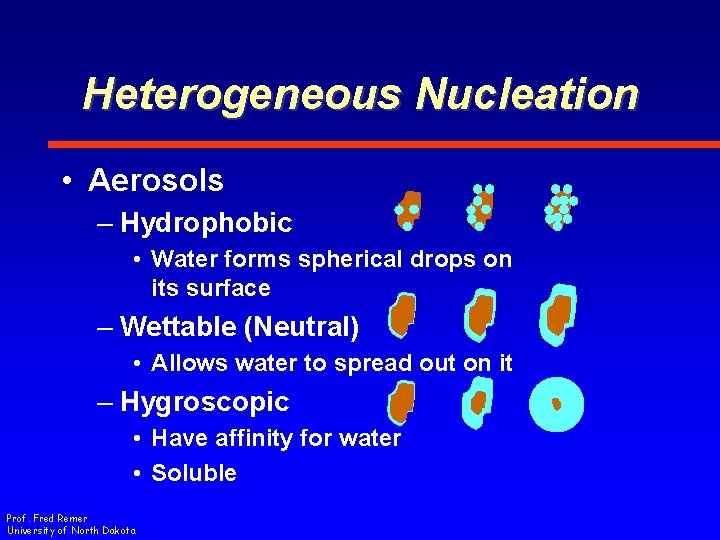
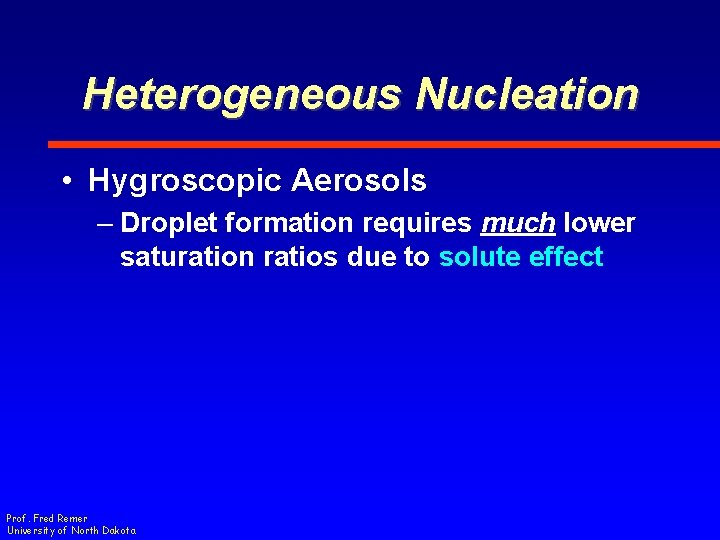
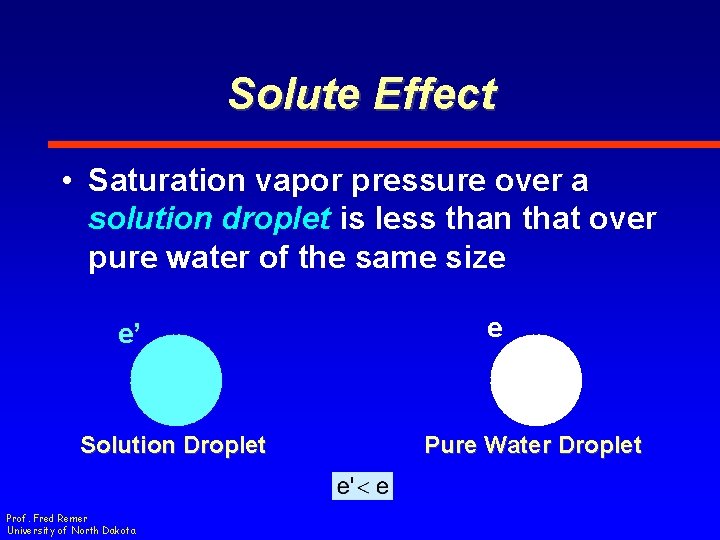
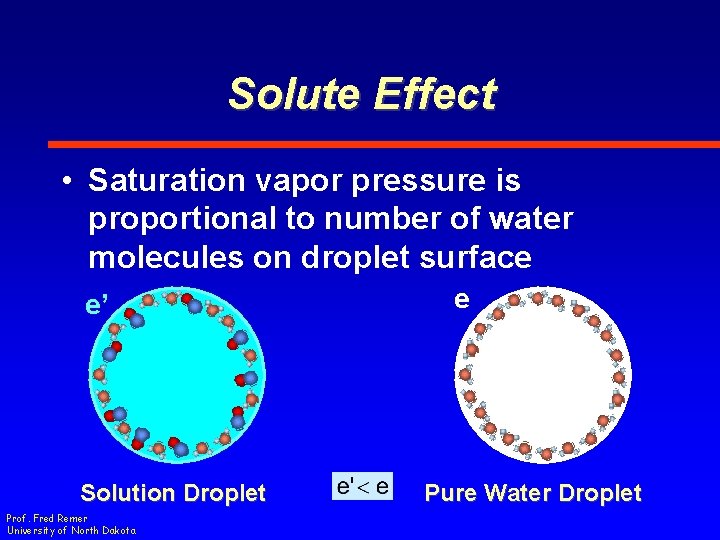
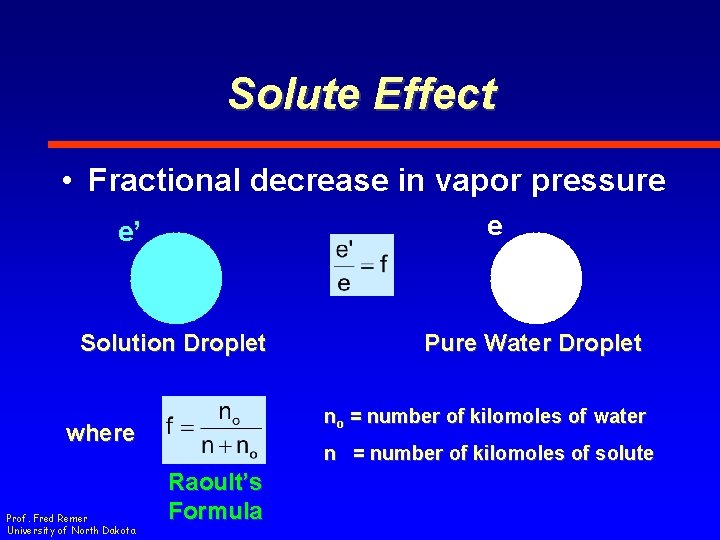
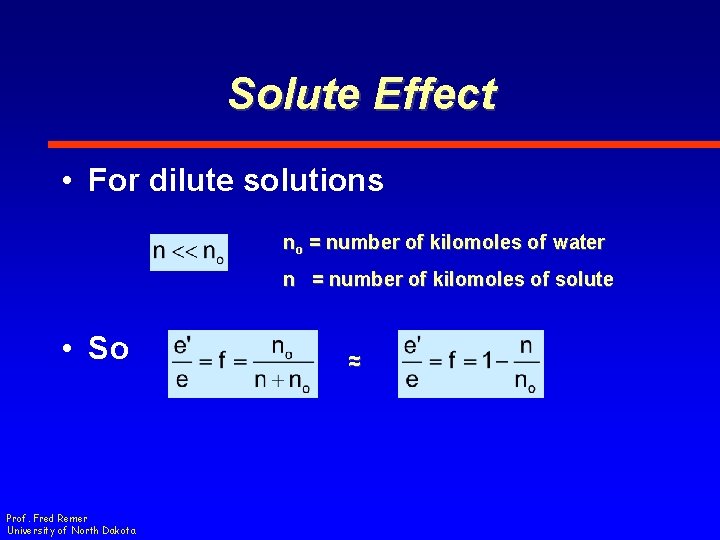
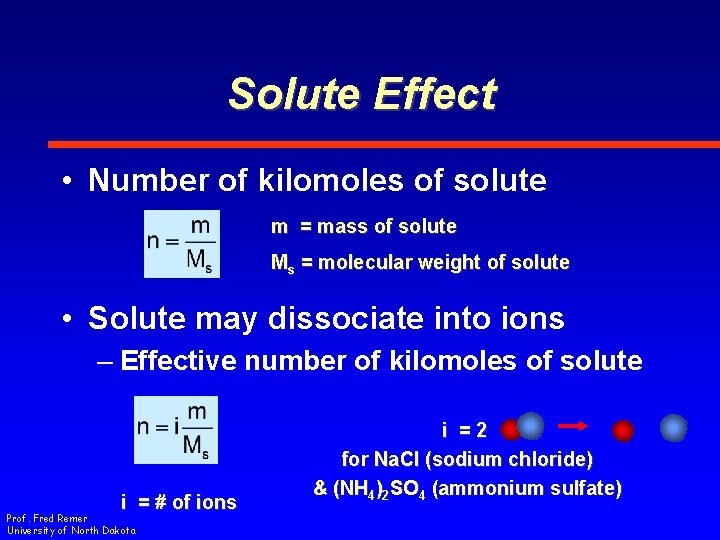
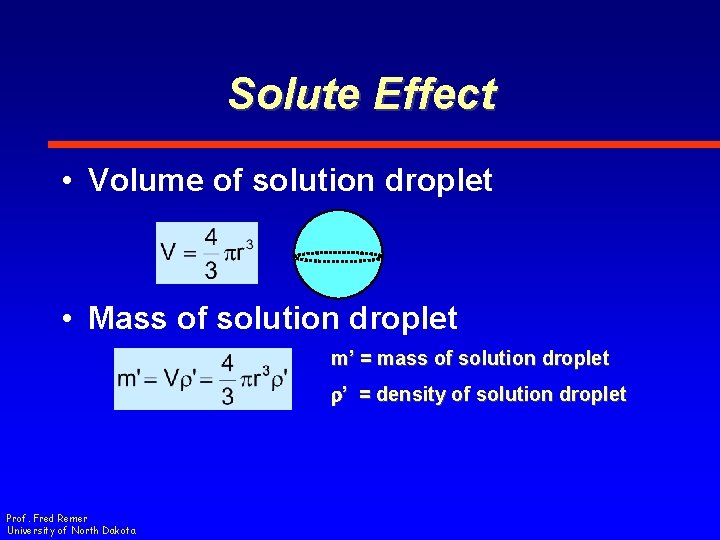
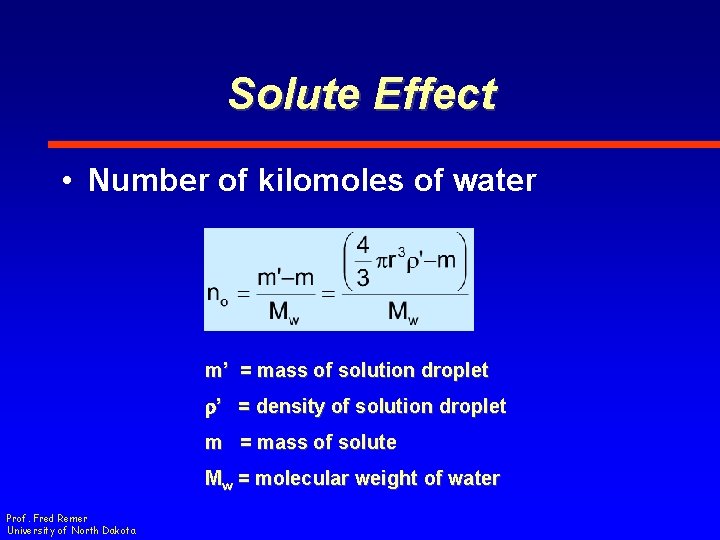
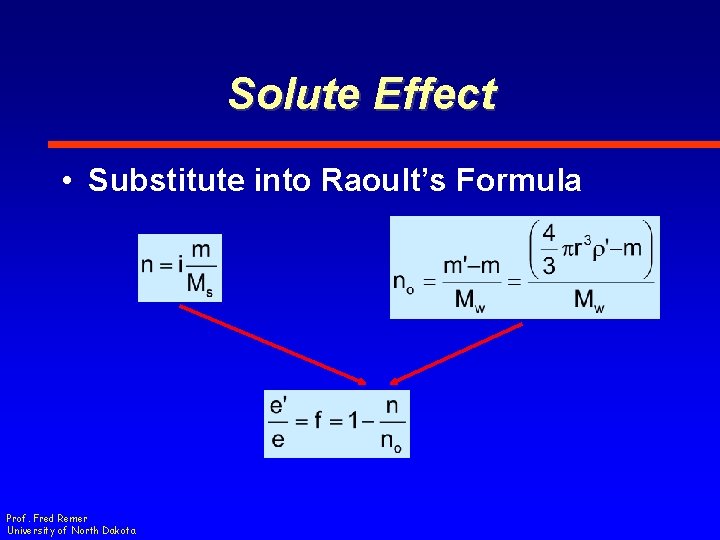
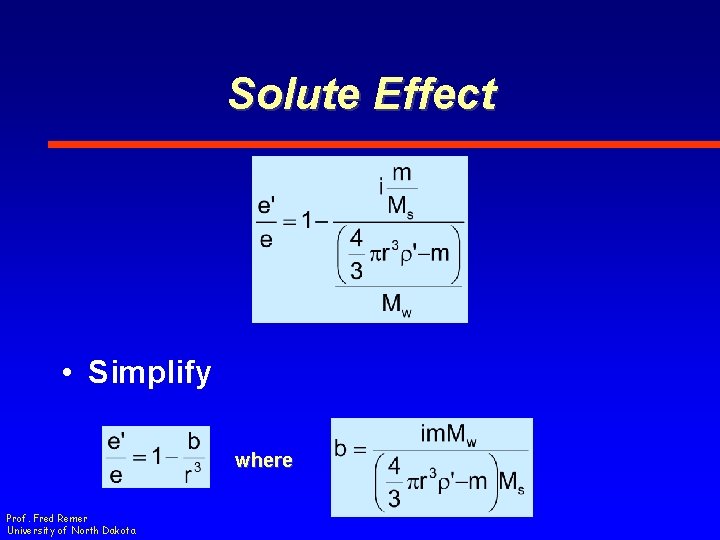
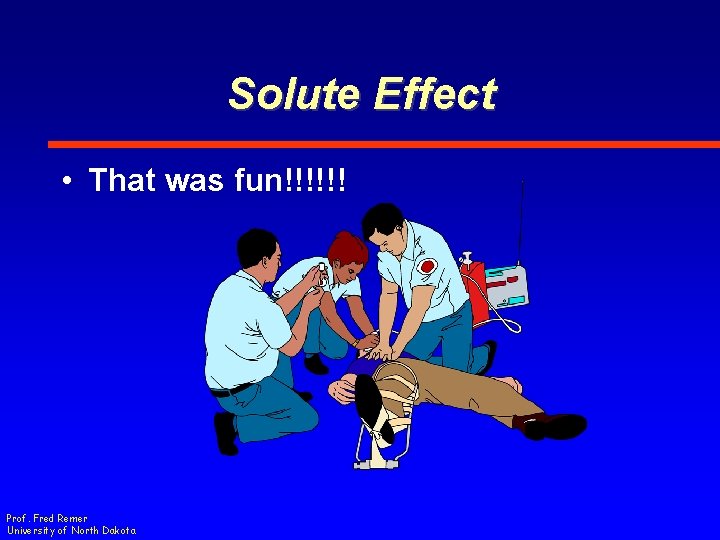
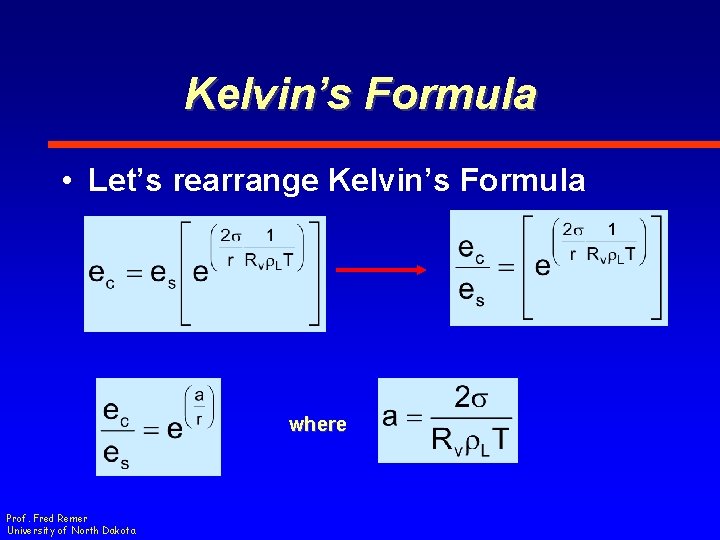
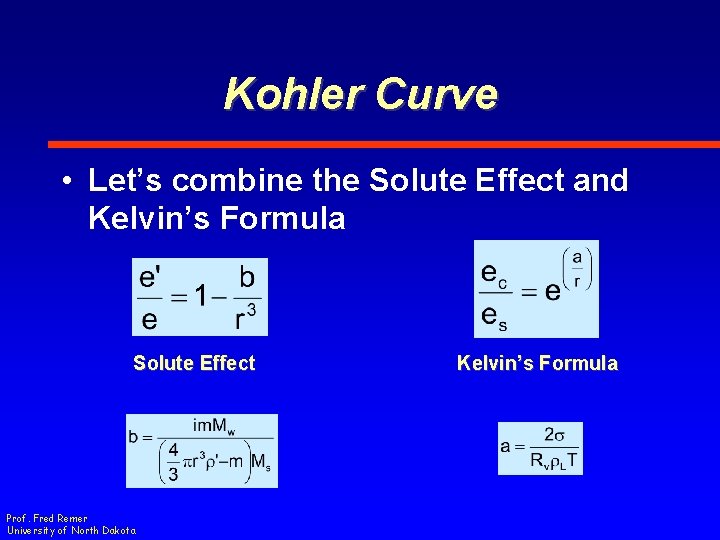
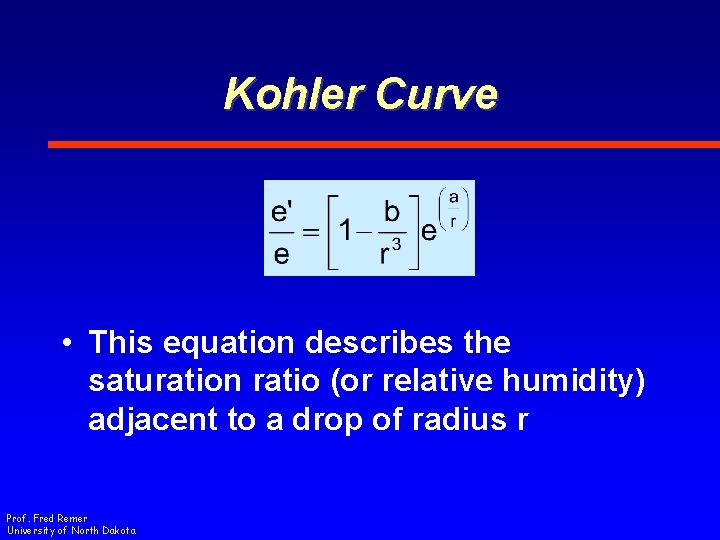
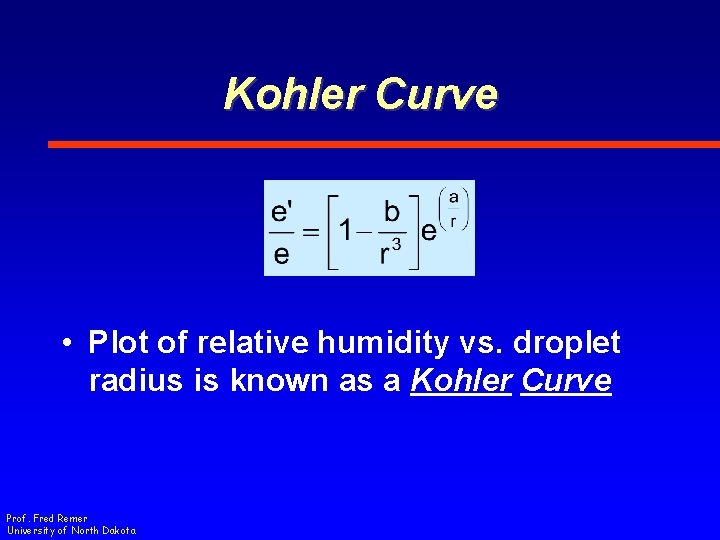
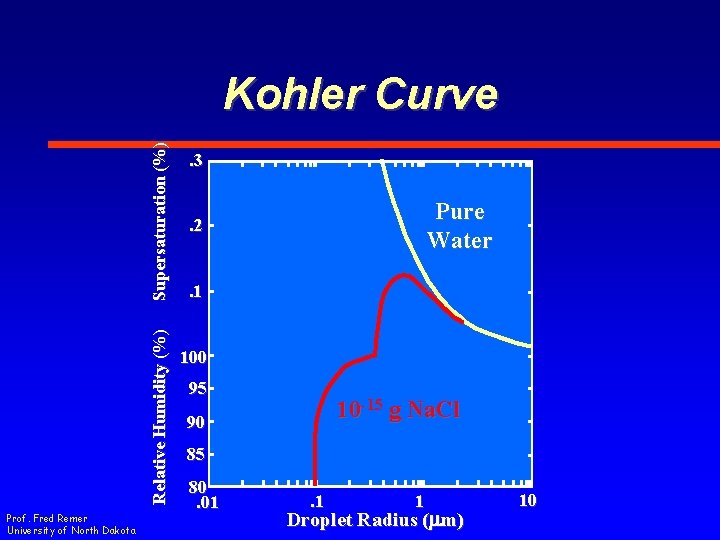
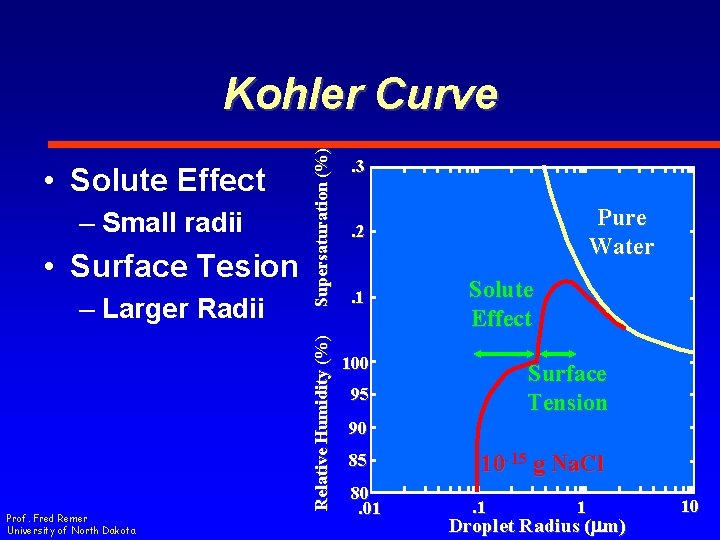
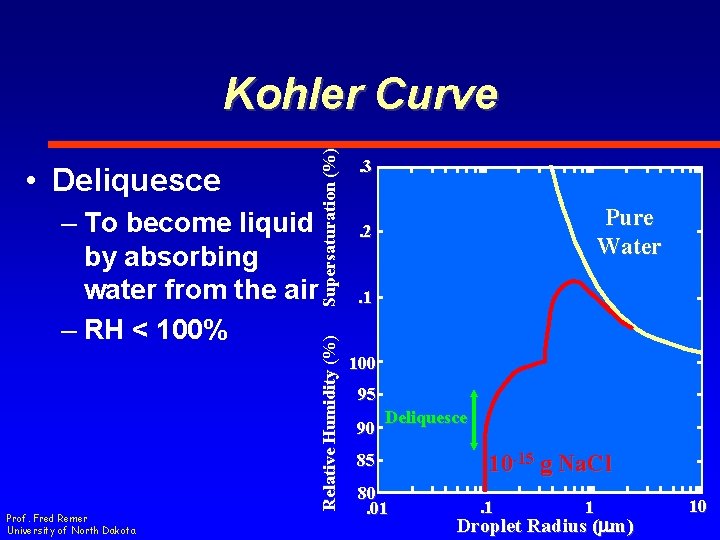
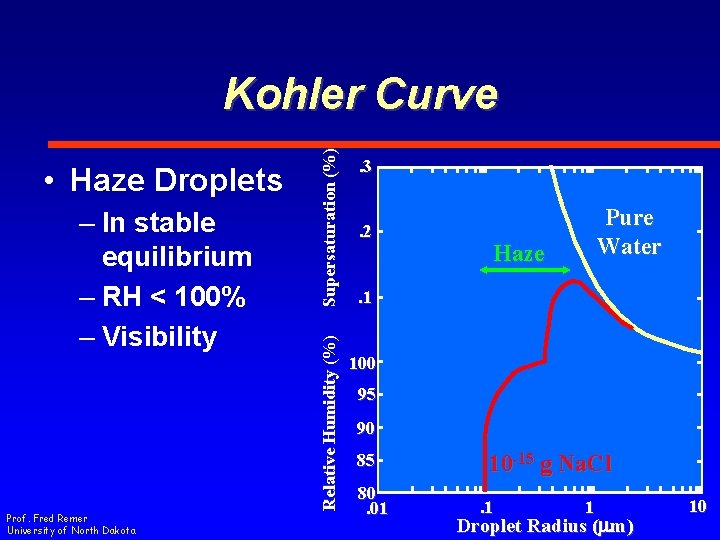
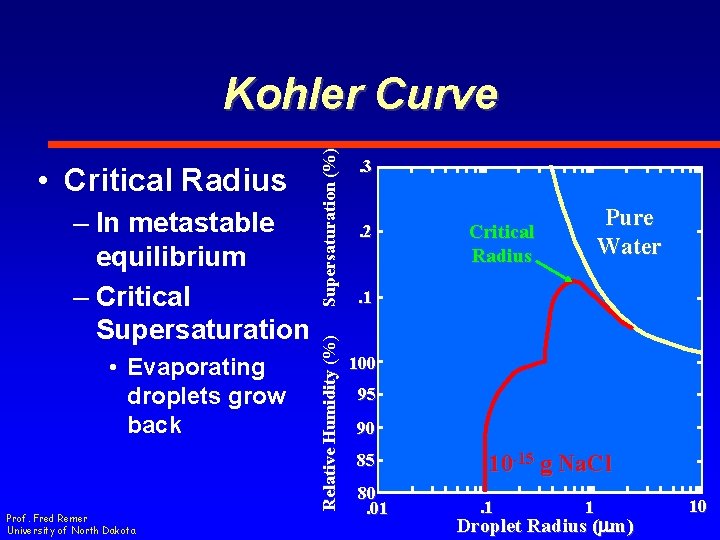
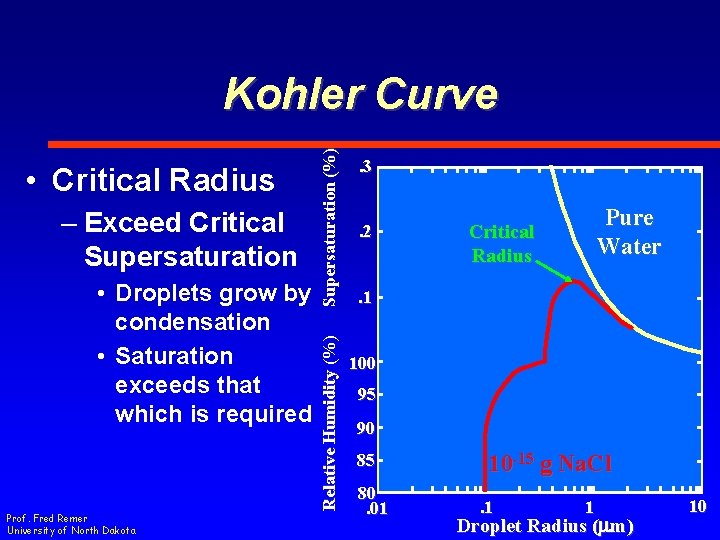
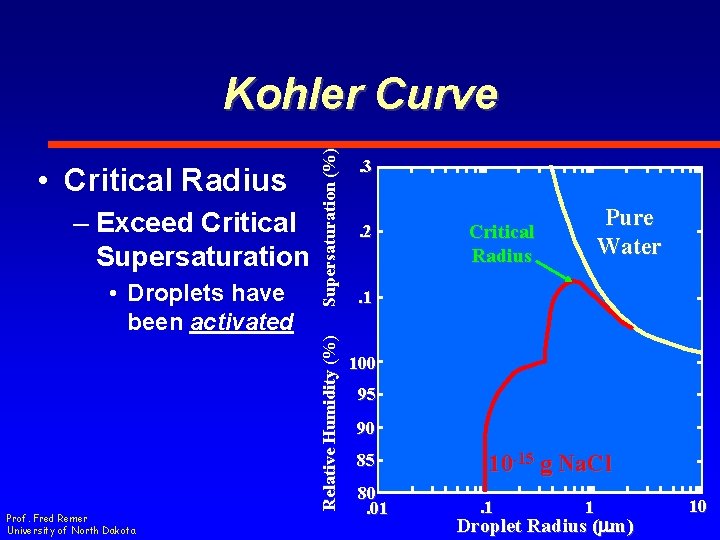
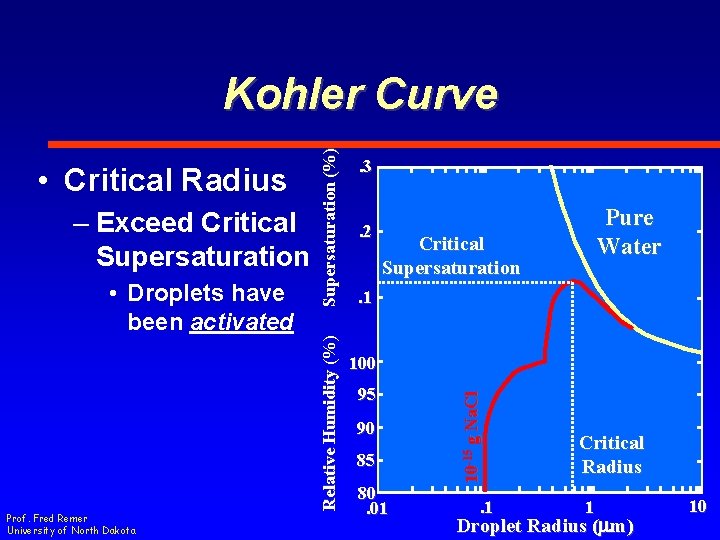
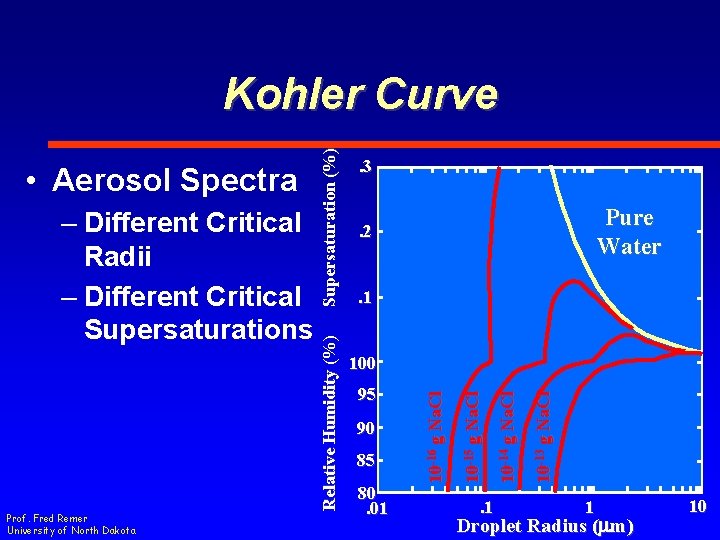
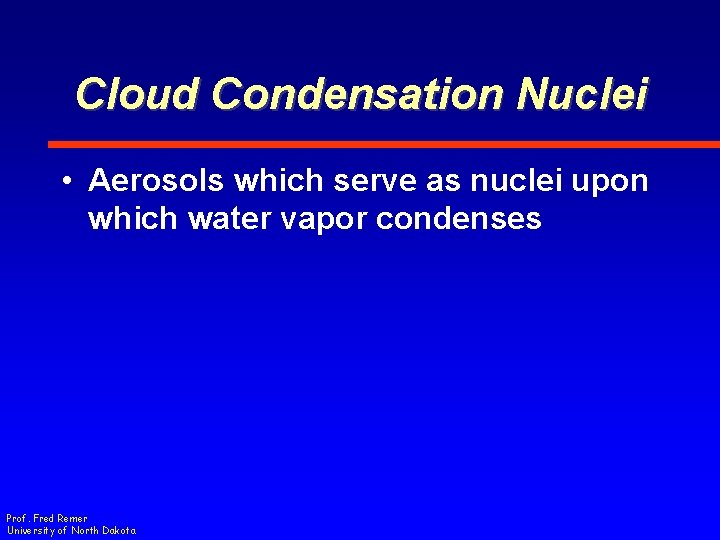
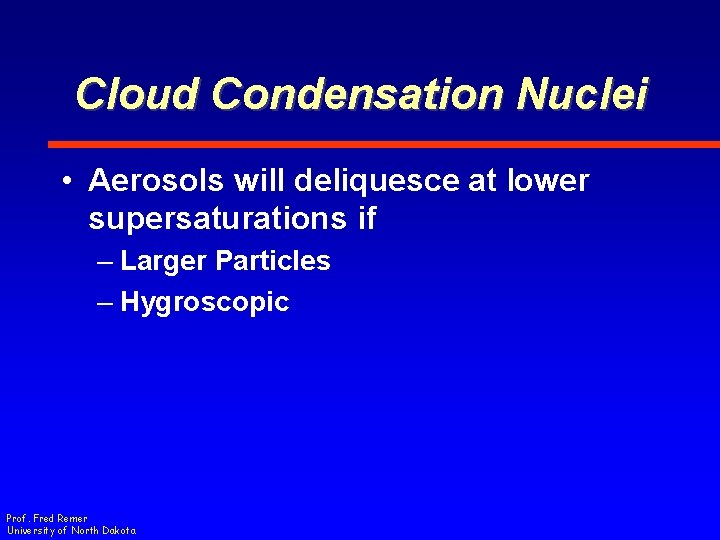
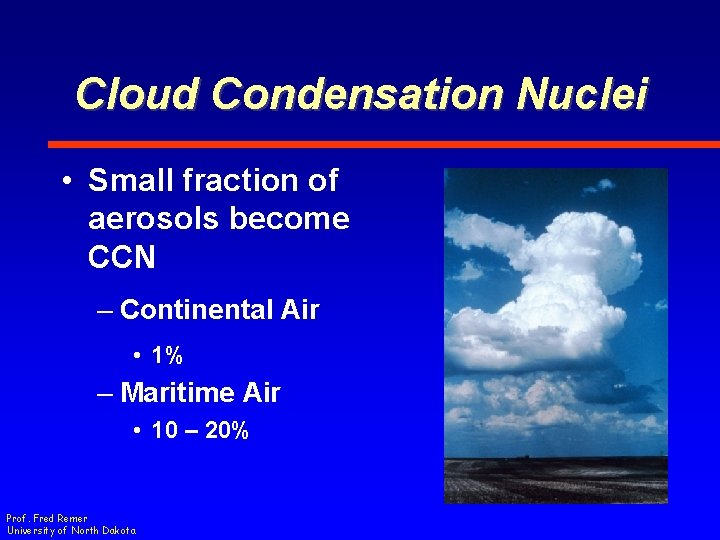
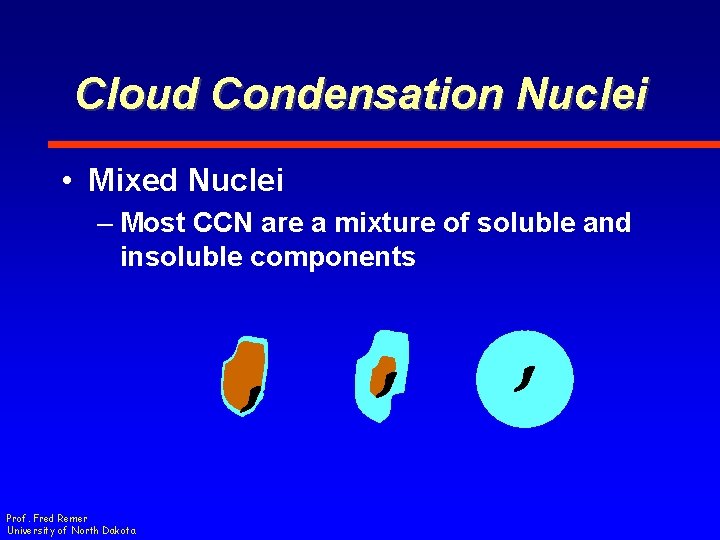
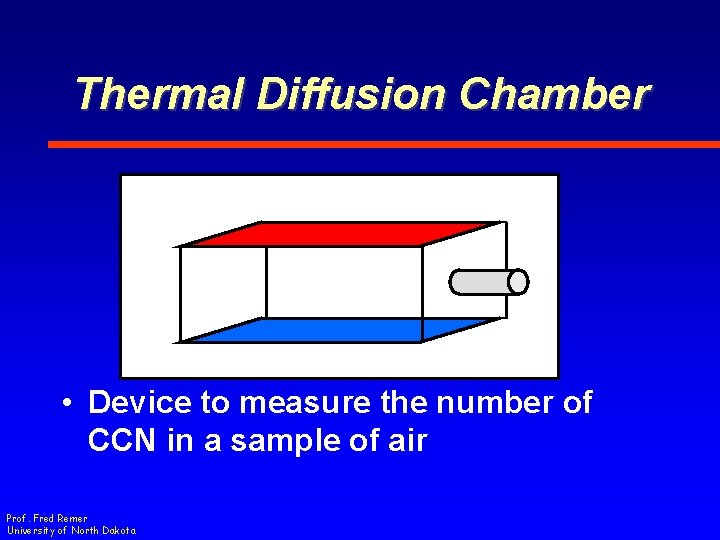
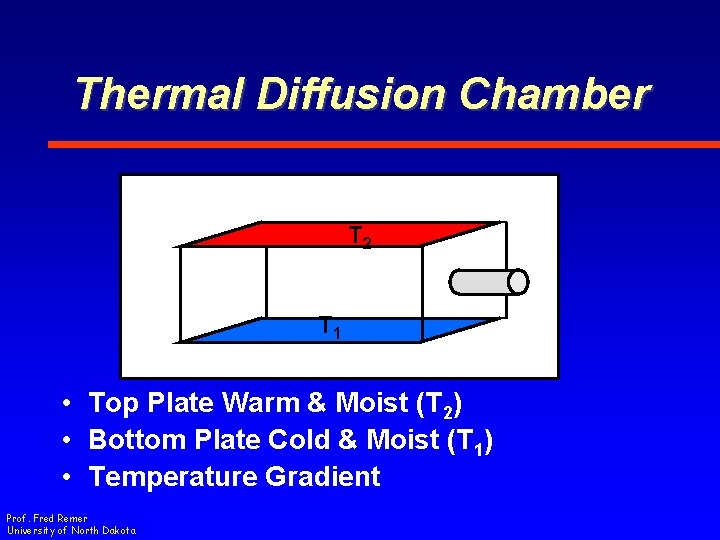
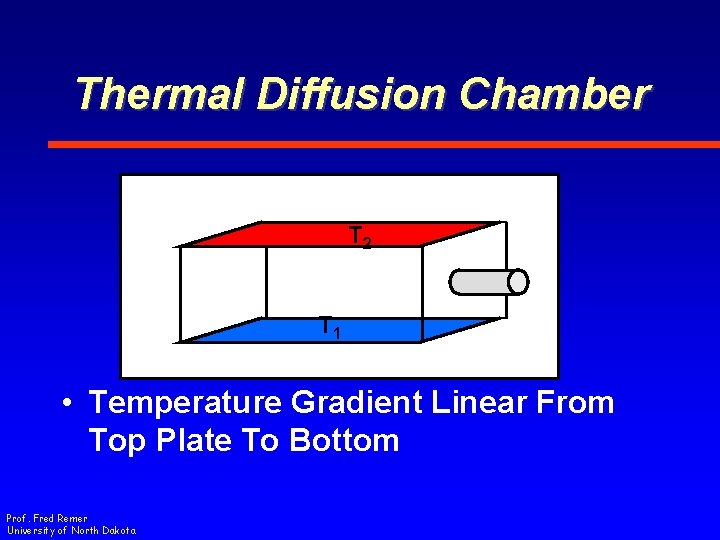
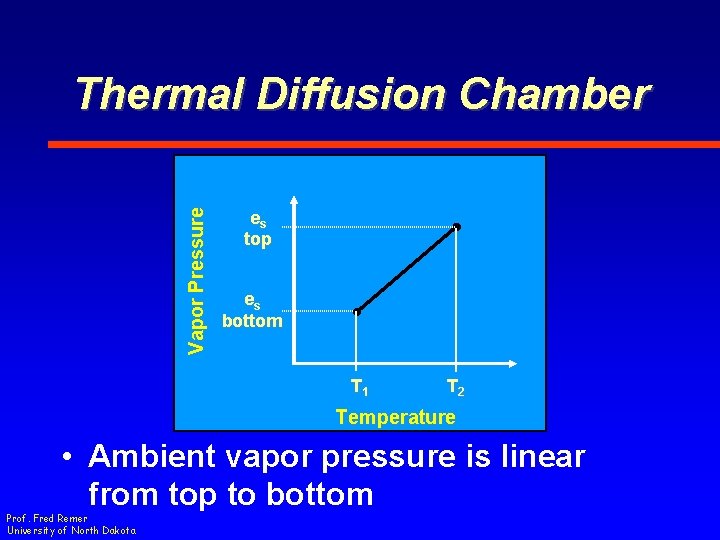
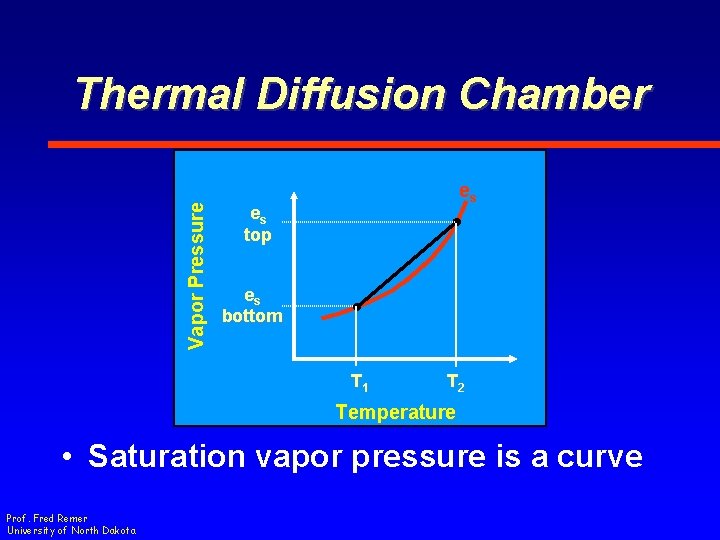
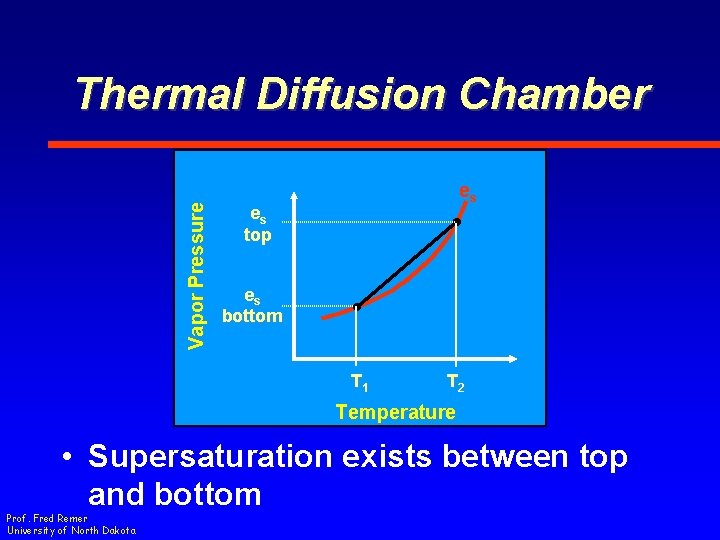
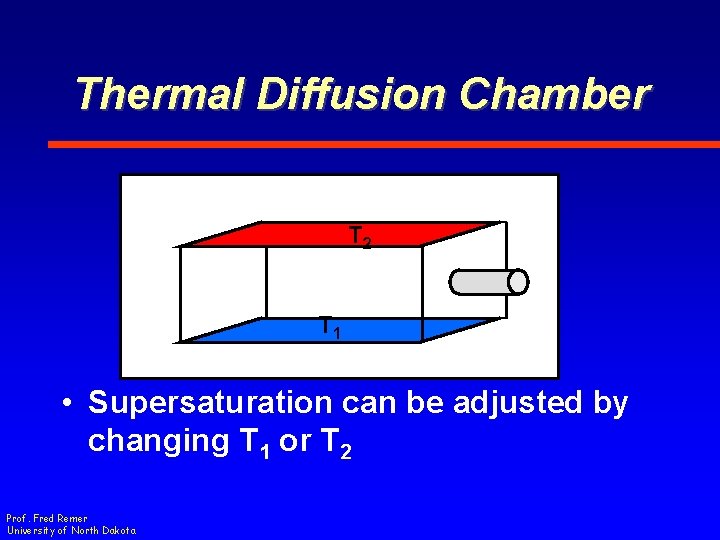
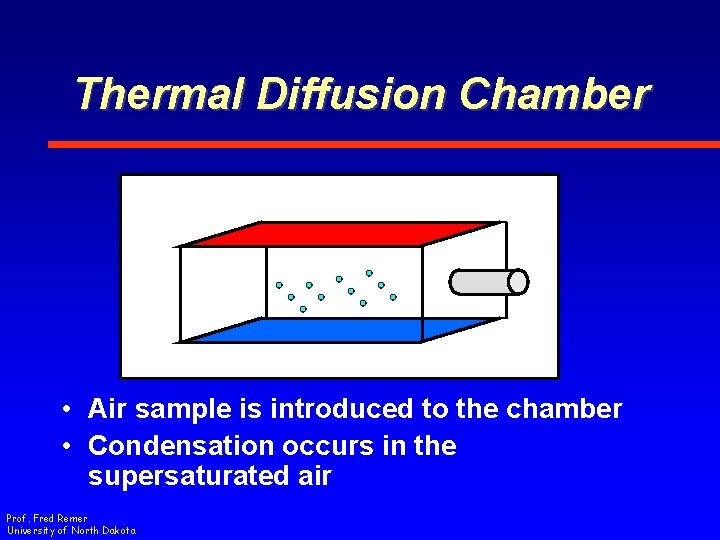
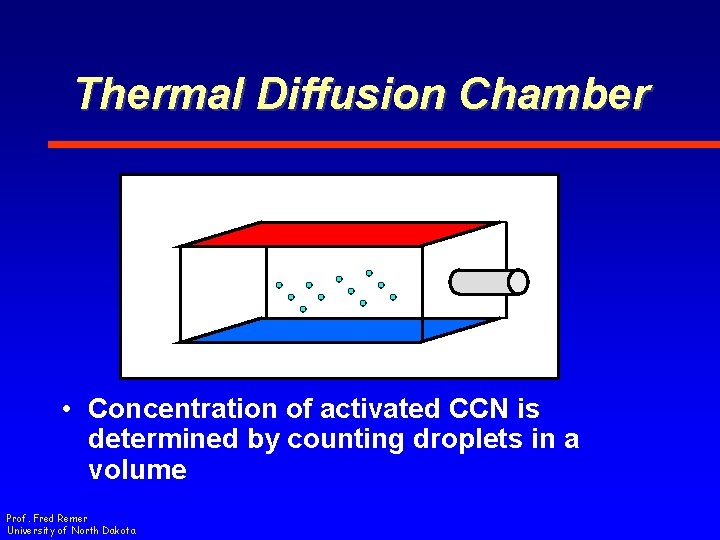
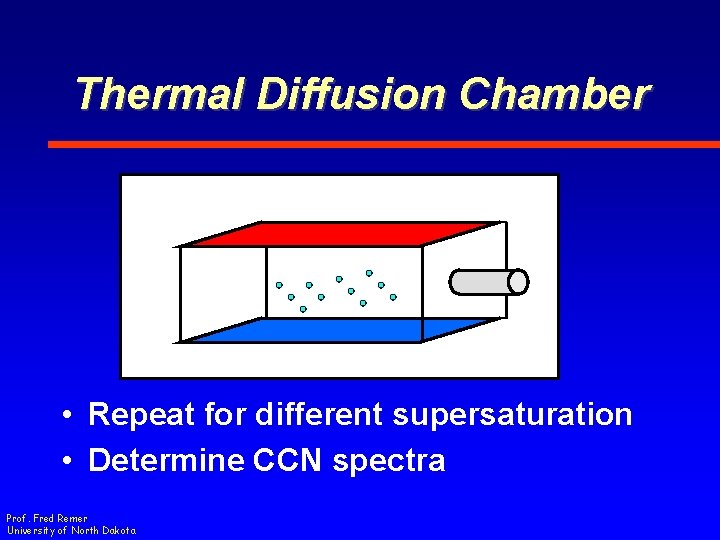
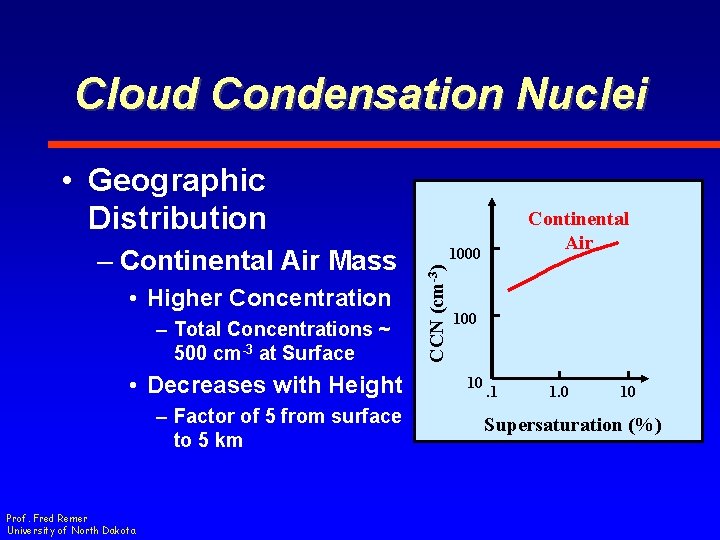
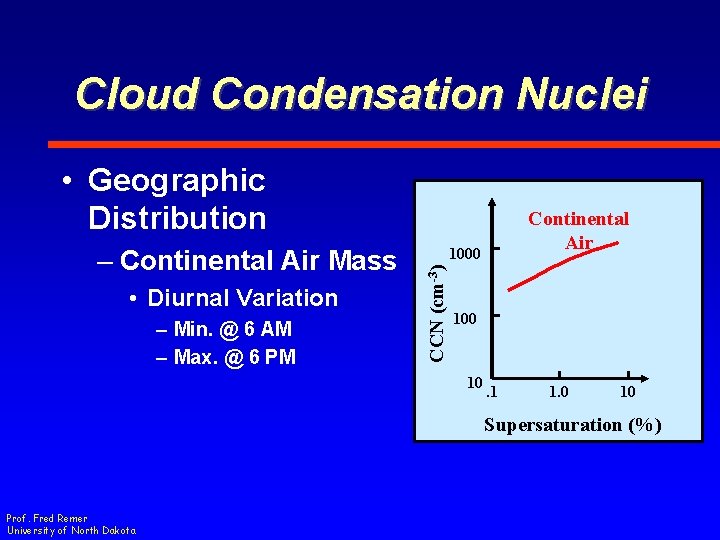
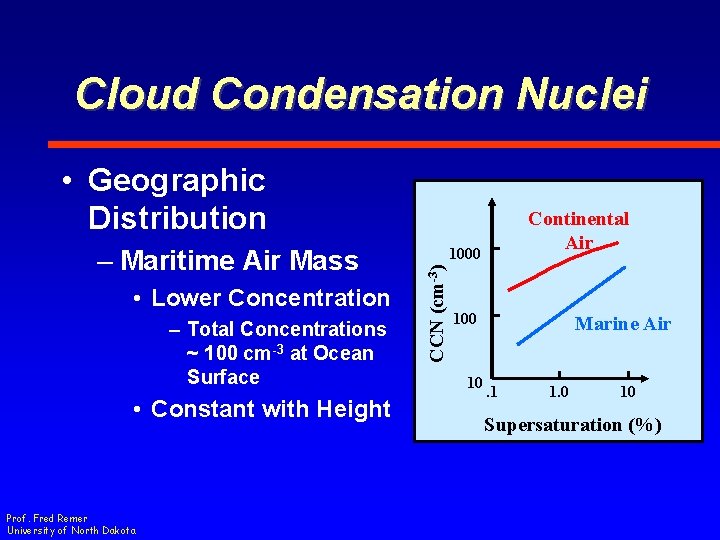
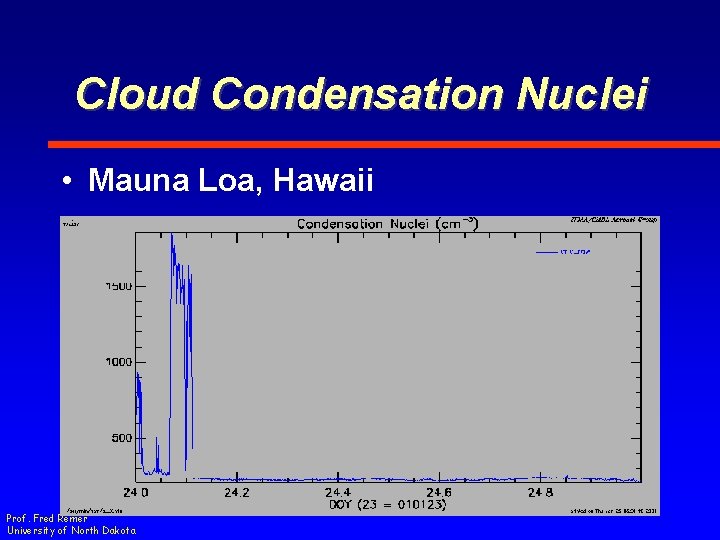
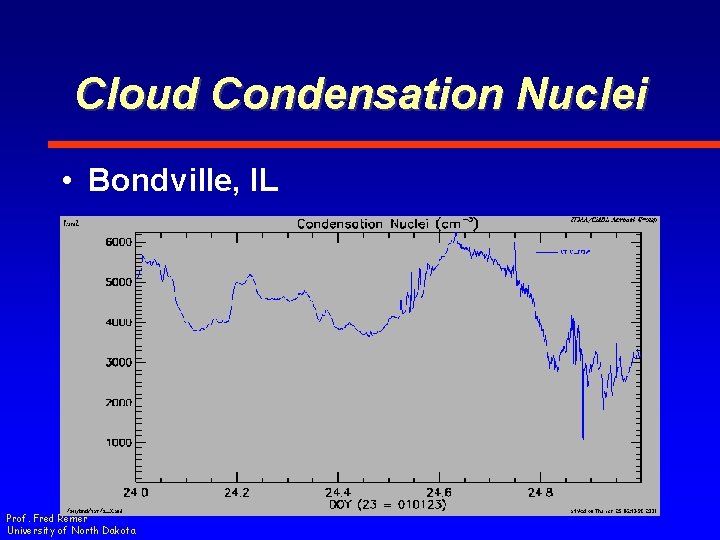
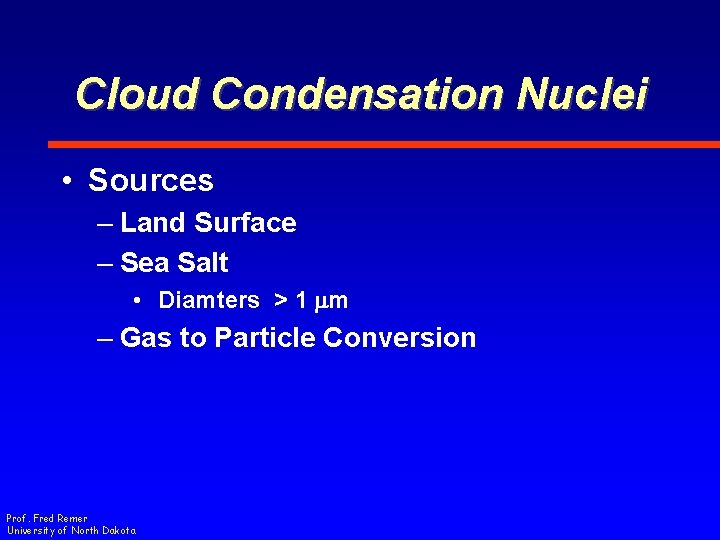
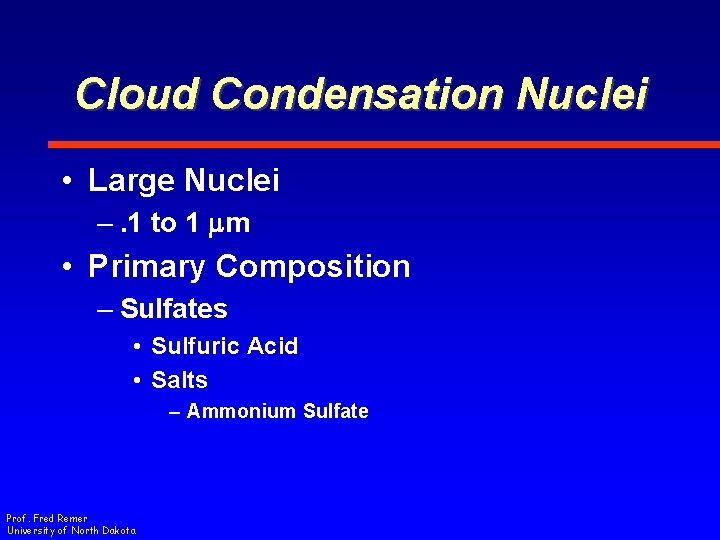
- Slides: 121
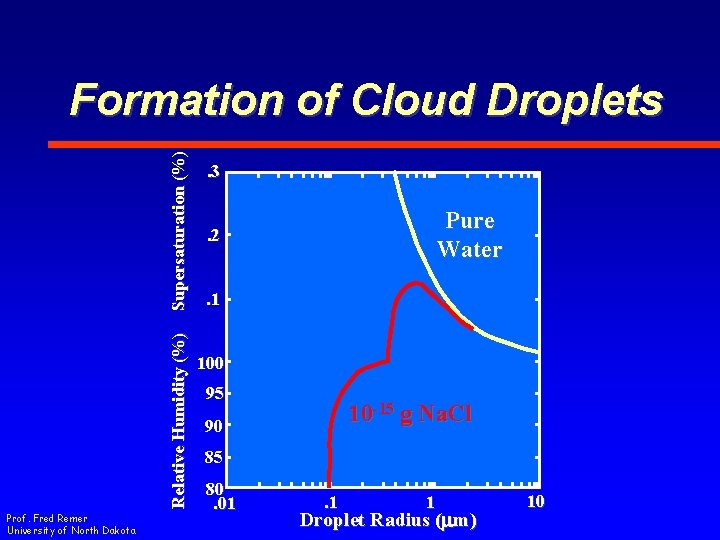
Relative Humidity (%) Supersaturation (%) Formation of Cloud Droplets Prof. Fred Remer University of North Dakota . 3 Pure Water . 2. 1 100 95 10 -15 g Na. Cl 90 85 80. 01 . 1 1 Droplet Radius (mm) 10
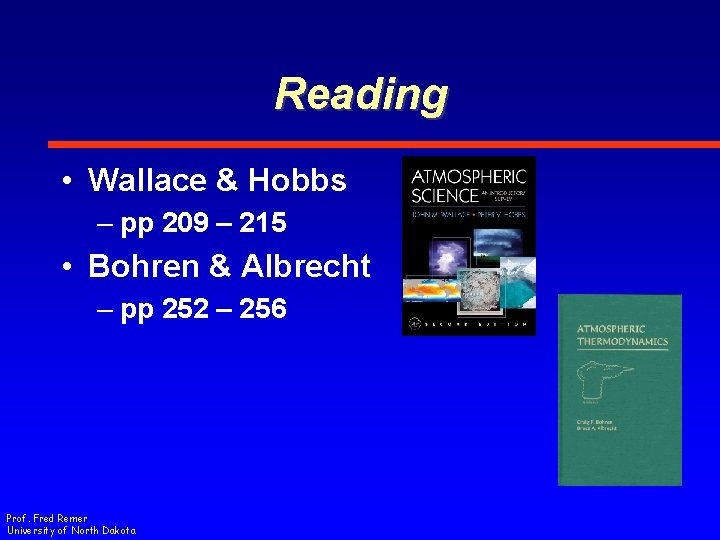
Reading • Wallace & Hobbs – pp 209 – 215 • Bohren & Albrecht – pp 252 – 256 Prof. Fred Remer University of North Dakota
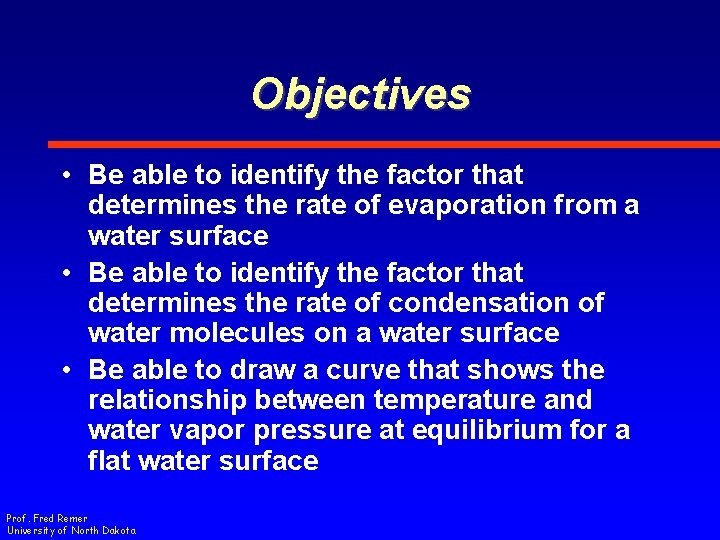
Objectives • Be able to identify the factor that determines the rate of evaporation from a water surface • Be able to identify the factor that determines the rate of condensation of water molecules on a water surface • Be able to draw a curve that shows the relationship between temperature and water vapor pressure at equilibrium for a flat water surface Prof. Fred Remer University of North Dakota
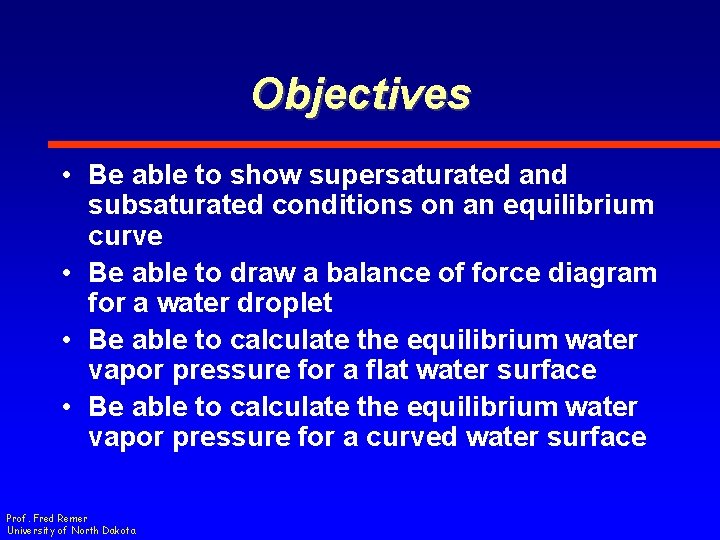
Objectives • Be able to show supersaturated and subsaturated conditions on an equilibrium curve • Be able to draw a balance of force diagram for a water droplet • Be able to calculate the equilibrium water vapor pressure for a flat water surface • Be able to calculate the equilibrium water vapor pressure for a curved water surface Prof. Fred Remer University of North Dakota
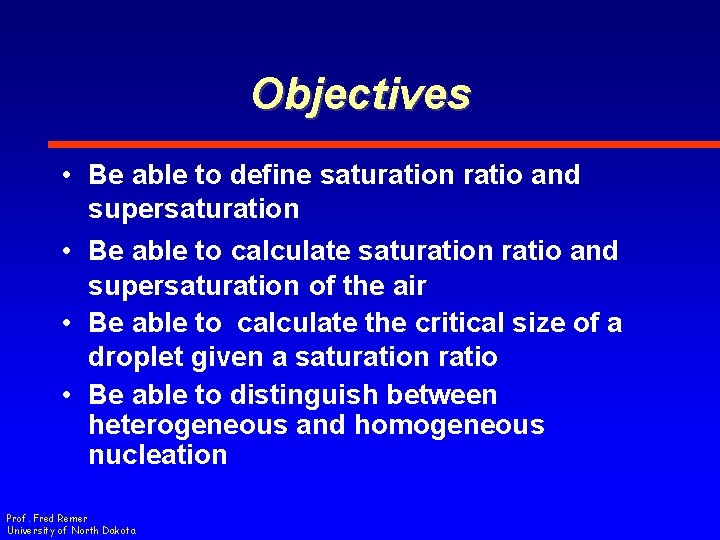
Objectives • Be able to define saturation ratio and supersaturation • Be able to calculate saturation ratio and supersaturation of the air • Be able to calculate the critical size of a droplet given a saturation ratio • Be able to distinguish between heterogeneous and homogeneous nucleation Prof. Fred Remer University of North Dakota
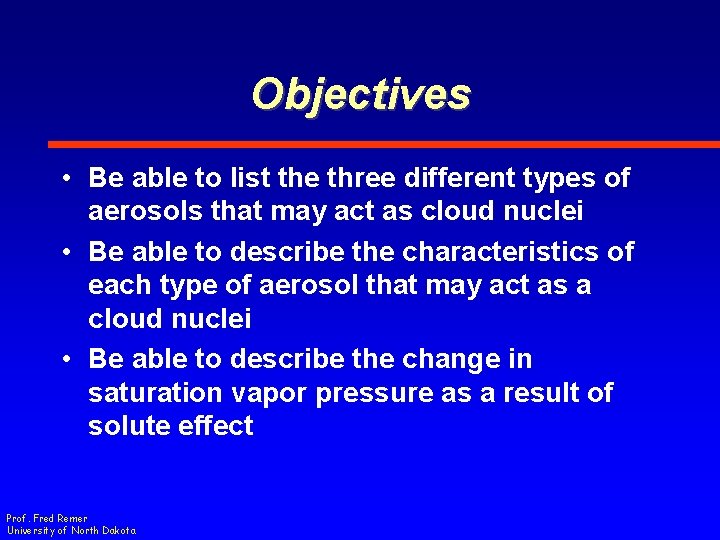
Objectives • Be able to list the three different types of aerosols that may act as cloud nuclei • Be able to describe the characteristics of each type of aerosol that may act as a cloud nuclei • Be able to describe the change in saturation vapor pressure as a result of solute effect Prof. Fred Remer University of North Dakota
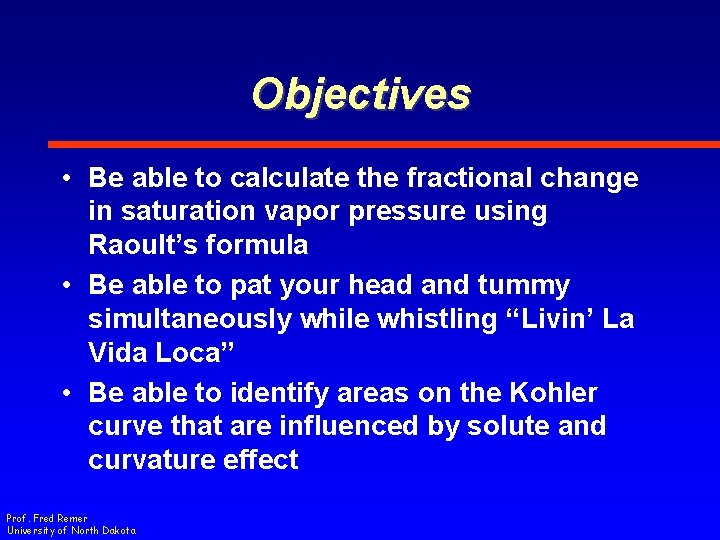
Objectives • Be able to calculate the fractional change in saturation vapor pressure using Raoult’s formula • Be able to pat your head and tummy simultaneously while whistling “Livin’ La Vida Loca” • Be able to identify areas on the Kohler curve that are influenced by solute and curvature effect Prof. Fred Remer University of North Dakota
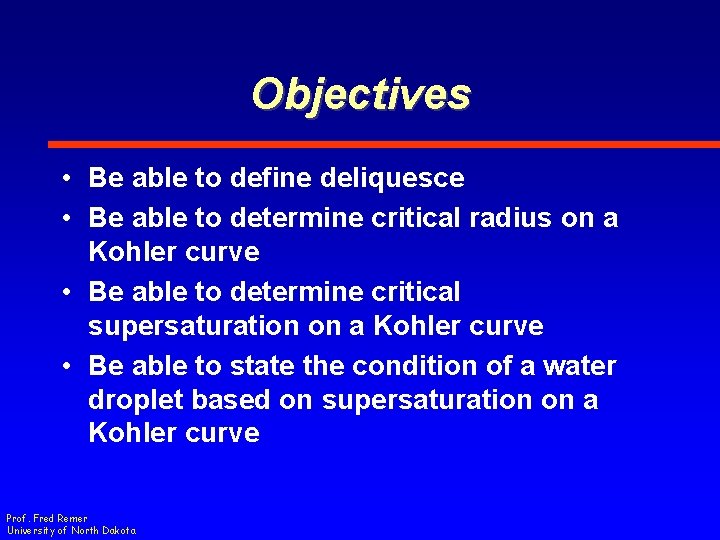
Objectives • Be able to define deliquesce • Be able to determine critical radius on a Kohler curve • Be able to determine critical supersaturation on a Kohler curve • Be able to state the condition of a water droplet based on supersaturation on a Kohler curve Prof. Fred Remer University of North Dakota
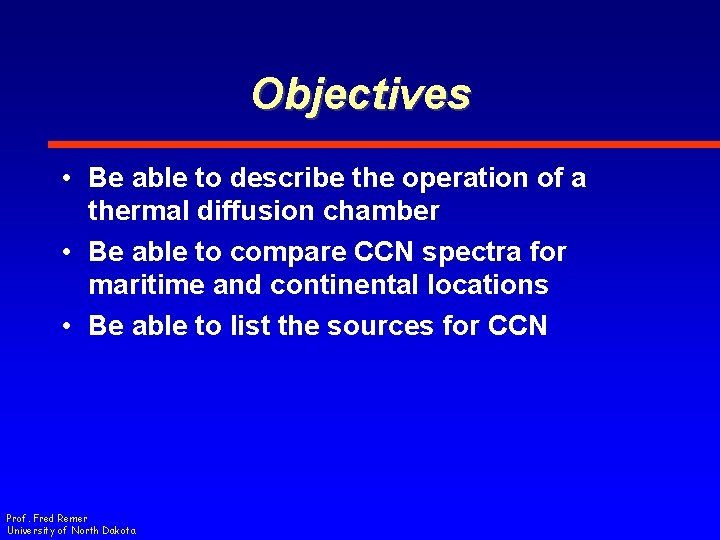
Objectives • Be able to describe the operation of a thermal diffusion chamber • Be able to compare CCN spectra for maritime and continental locations • Be able to list the sources for CCN Prof. Fred Remer University of North Dakota
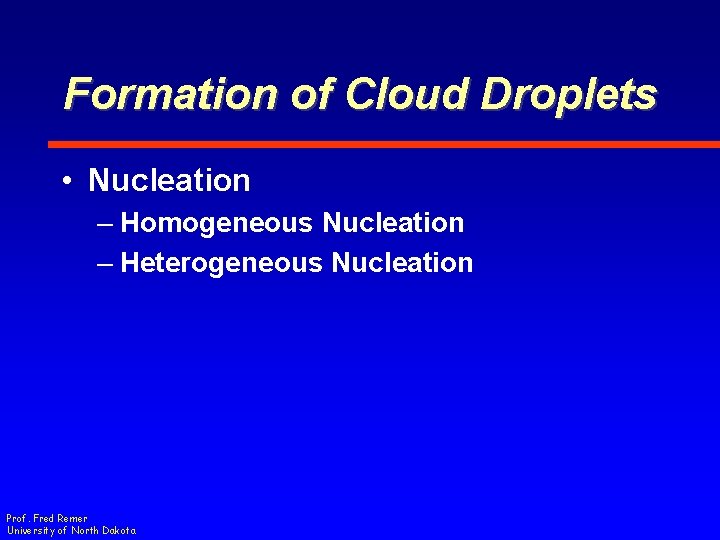
Formation of Cloud Droplets • Nucleation – Homogeneous Nucleation – Heterogeneous Nucleation Prof. Fred Remer University of North Dakota
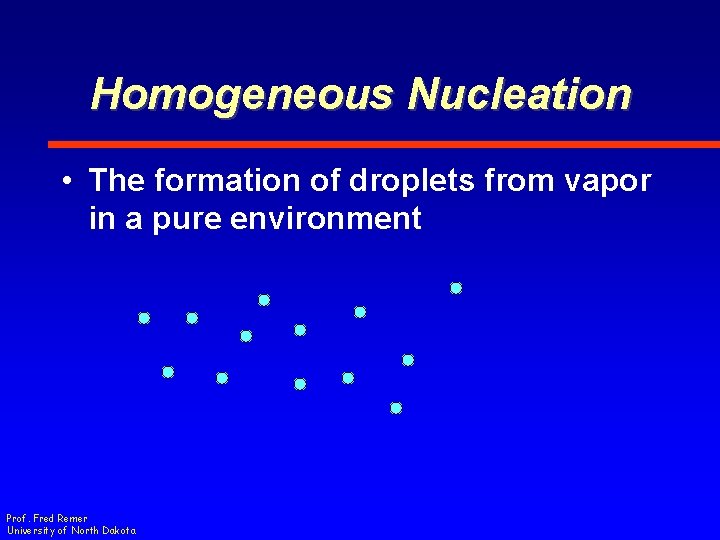
Homogeneous Nucleation • The formation of droplets from vapor in a pure environment Prof. Fred Remer University of North Dakota
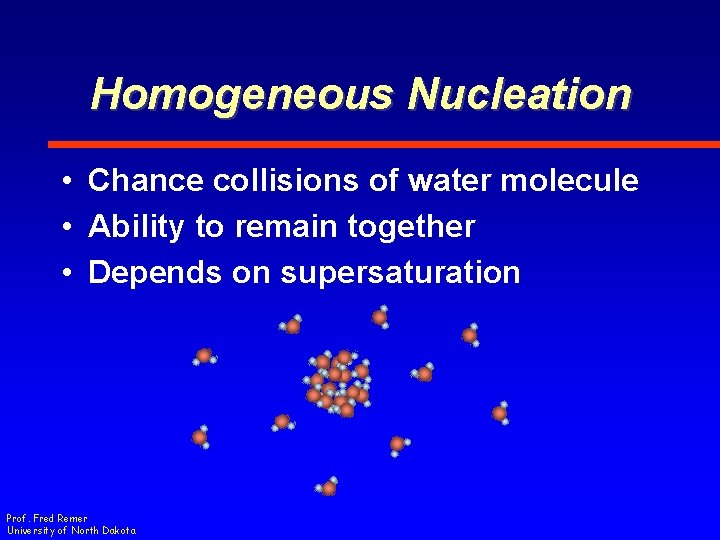
Homogeneous Nucleation • Chance collisions of water molecule • Ability to remain together • Depends on supersaturation Prof. Fred Remer University of North Dakota
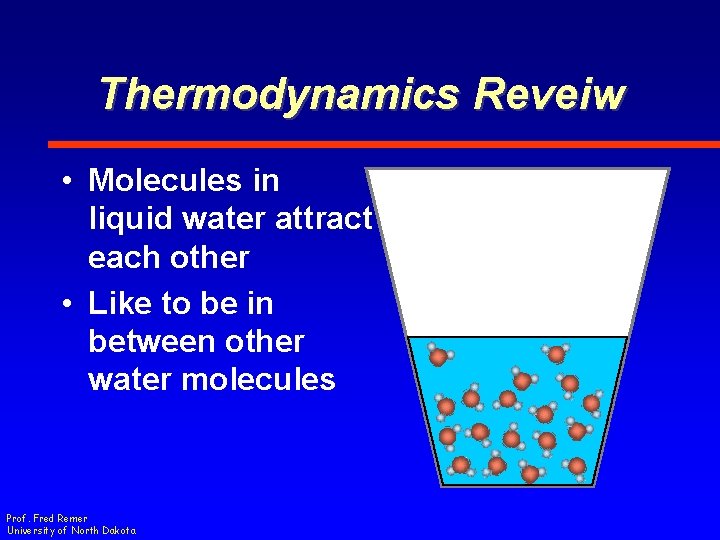
Thermodynamics Reveiw • Molecules in liquid water attract each other • Like to be in between other water molecules Prof. Fred Remer University of North Dakota
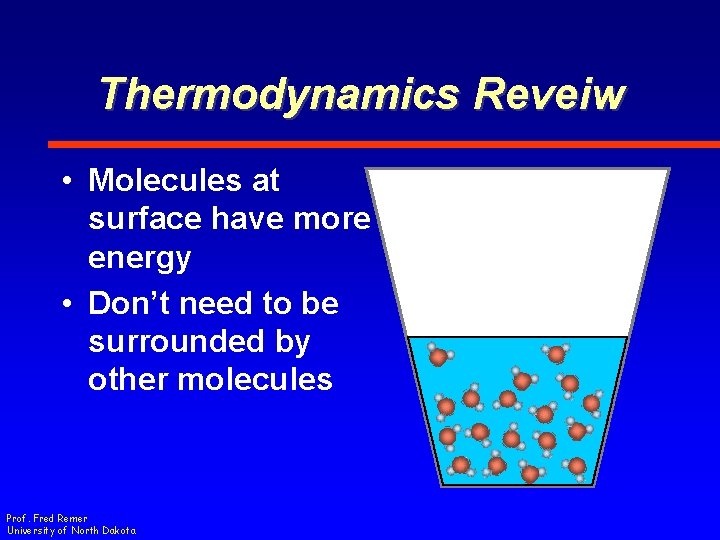
Thermodynamics Reveiw • Molecules at surface have more energy • Don’t need to be surrounded by other molecules Prof. Fred Remer University of North Dakota
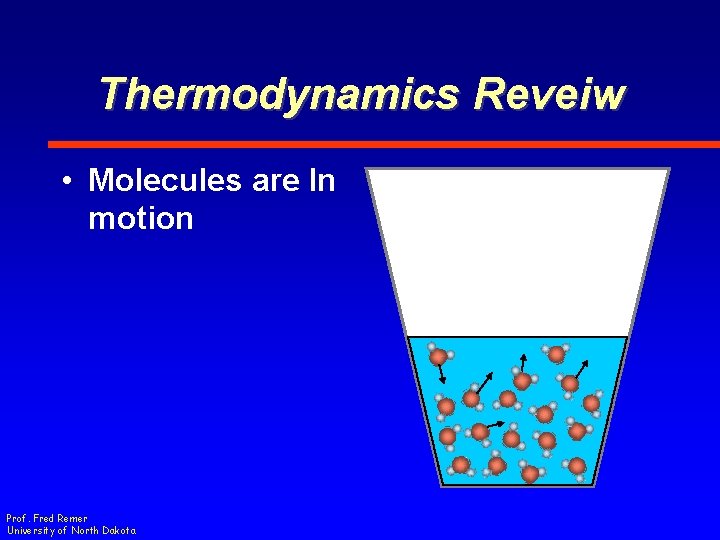
Thermodynamics Reveiw • Molecules are In motion Prof. Fred Remer University of North Dakota
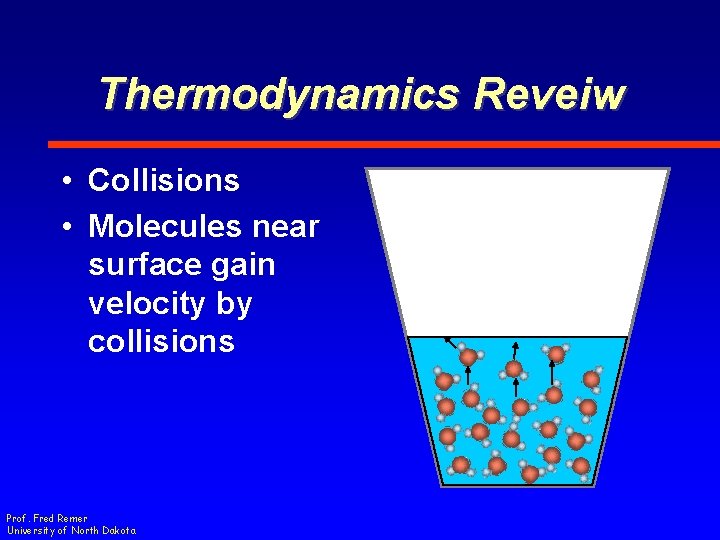
Thermodynamics Reveiw • Collisions • Molecules near surface gain velocity by collisions Prof. Fred Remer University of North Dakota
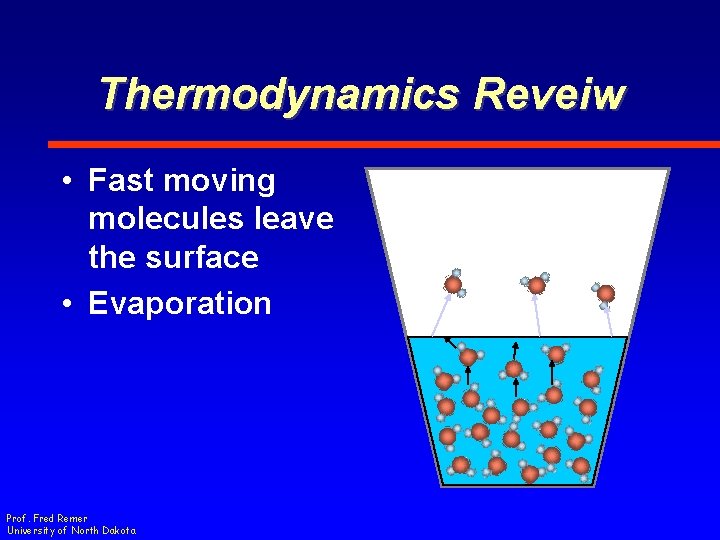
Thermodynamics Reveiw • Fast moving molecules leave the surface • Evaporation Prof. Fred Remer University of North Dakota
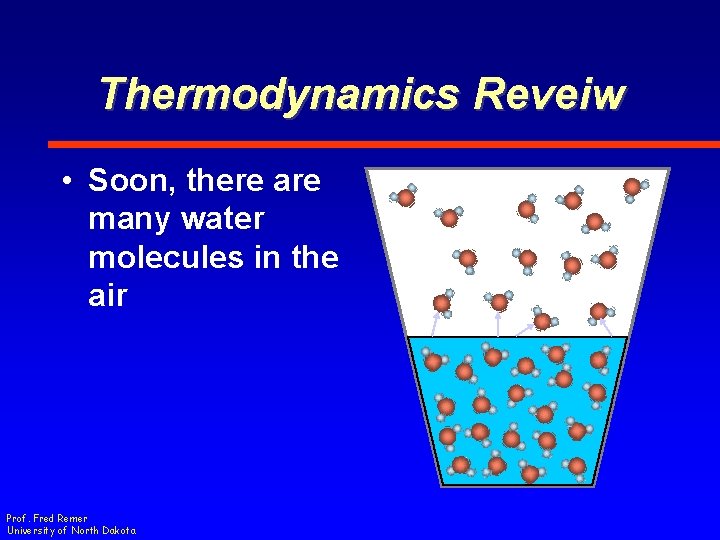
Thermodynamics Reveiw • Soon, there are many water molecules in the air Prof. Fred Remer University of North Dakota
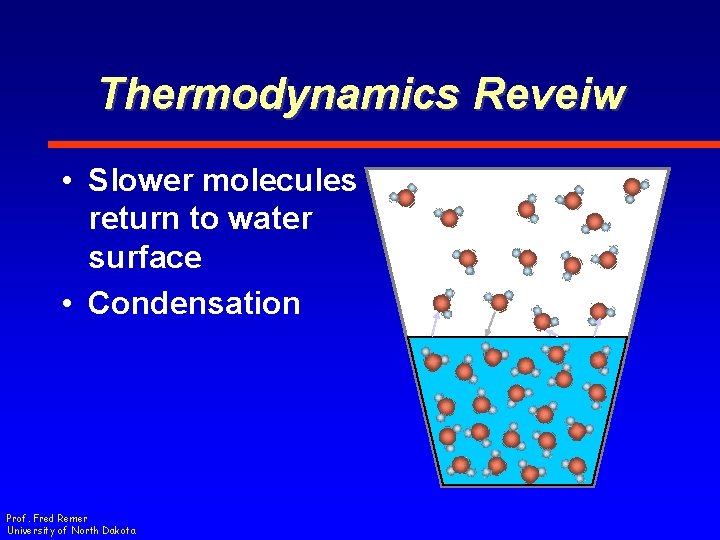
Thermodynamics Reveiw • Slower molecules return to water surface • Condensation Prof. Fred Remer University of North Dakota
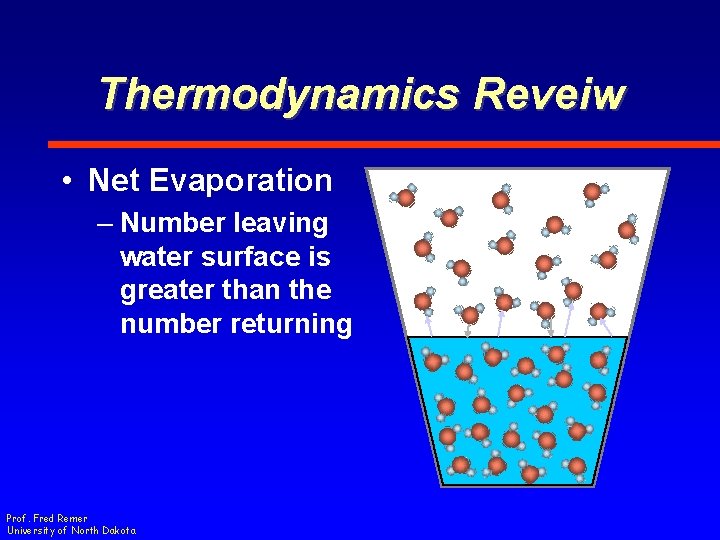
Thermodynamics Reveiw • Net Evaporation – Number leaving water surface is greater than the number returning Prof. Fred Remer University of North Dakota
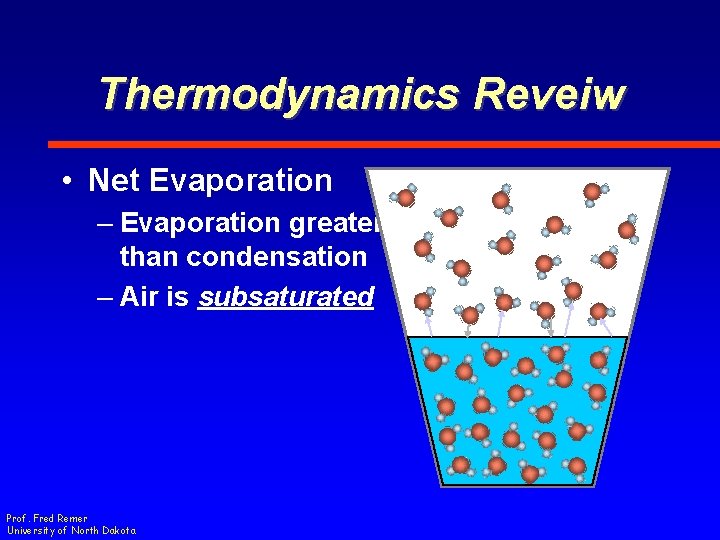
Thermodynamics Reveiw • Net Evaporation – Evaporation greater than condensation – Air is subsaturated Prof. Fred Remer University of North Dakota
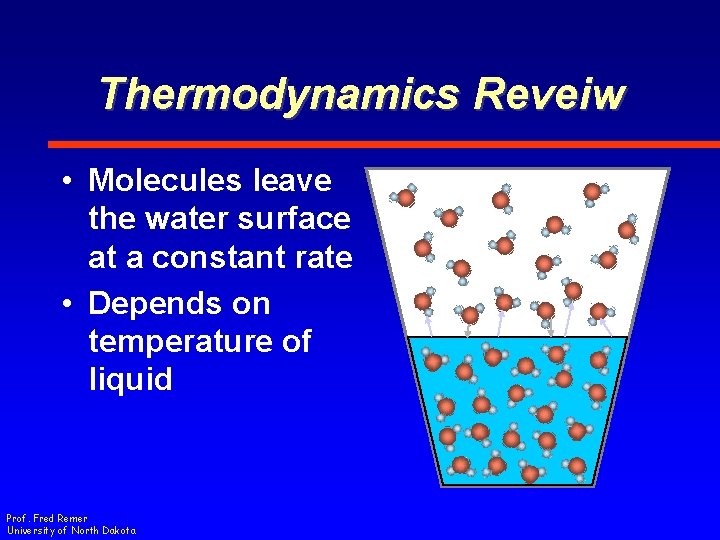
Thermodynamics Reveiw • Molecules leave the water surface at a constant rate • Depends on temperature of liquid Prof. Fred Remer University of North Dakota
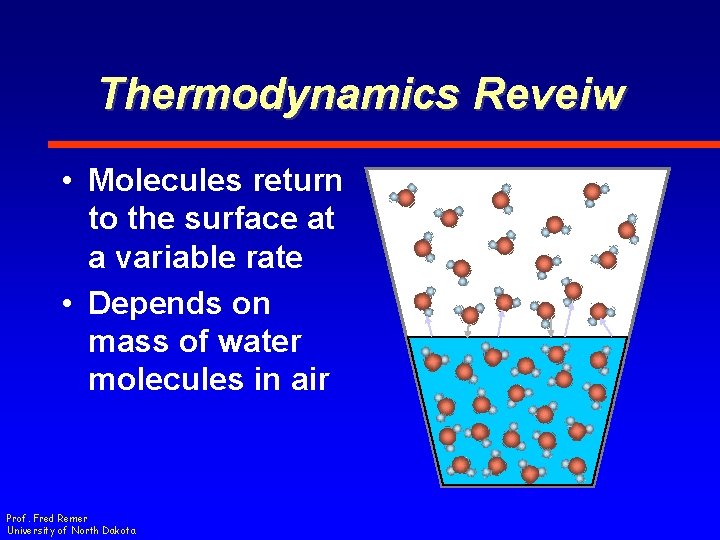
Thermodynamics Reveiw • Molecules return to the surface at a variable rate • Depends on mass of water molecules in air Prof. Fred Remer University of North Dakota
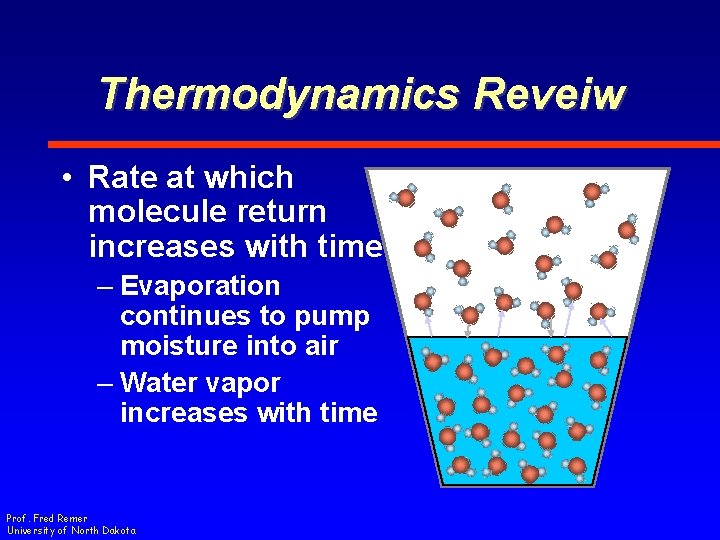
Thermodynamics Reveiw • Rate at which molecule return increases with time – Evaporation continues to pump moisture into air – Water vapor increases with time Prof. Fred Remer University of North Dakota
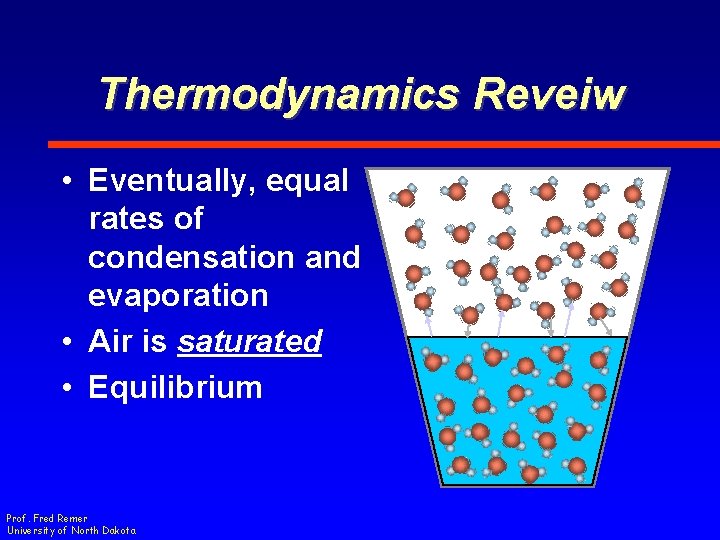
Thermodynamics Reveiw • Eventually, equal rates of condensation and evaporation • Air is saturated • Equilibrium Prof. Fred Remer University of North Dakota
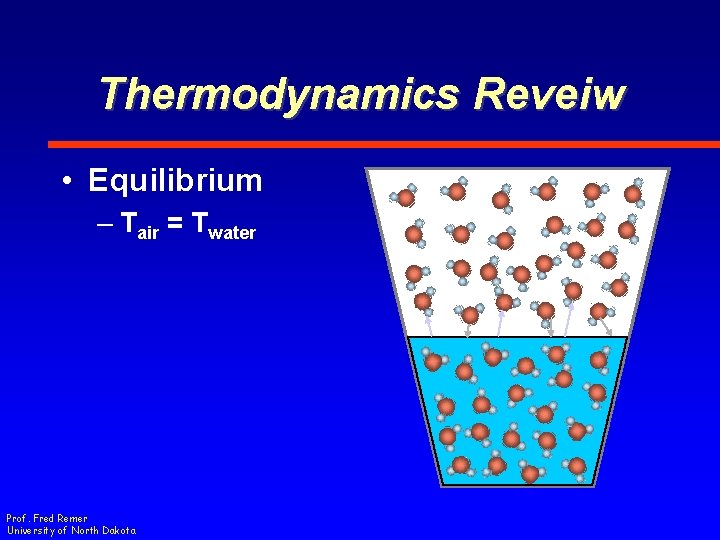
Thermodynamics Reveiw • Equilibrium – Tair = Twater Prof. Fred Remer University of North Dakota
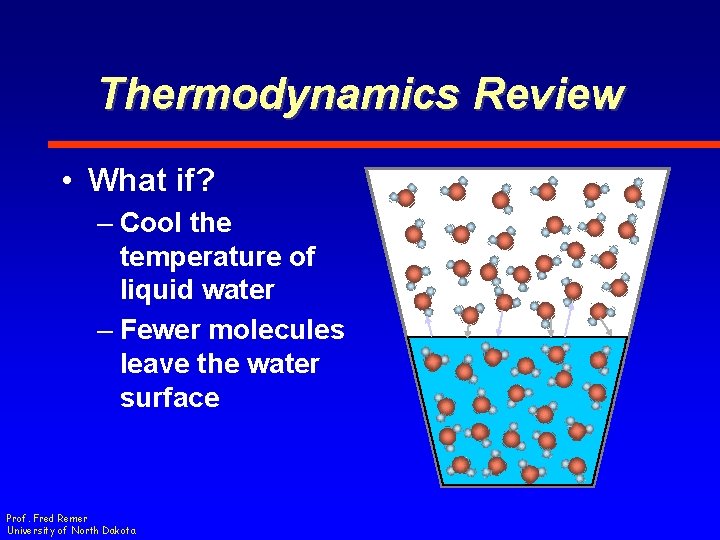
Thermodynamics Review • What if? – Cool the temperature of liquid water – Fewer molecules leave the water surface Prof. Fred Remer University of North Dakota
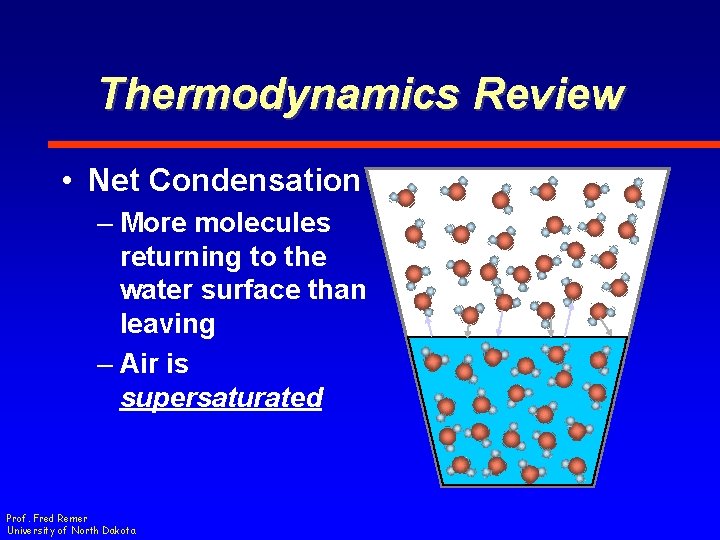
Thermodynamics Review • Net Condensation – More molecules returning to the water surface than leaving – Air is supersaturated Prof. Fred Remer University of North Dakota
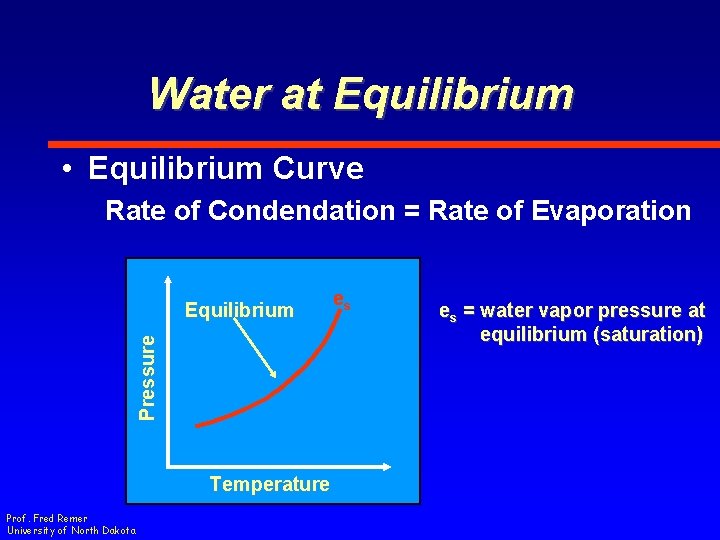
Water at Equilibrium • Equilibrium Curve Rate of Condendation = Rate of Evaporation Pressure Equilibrium Temperature Prof. Fred Remer University of North Dakota es es = water vapor pressure at equilibrium (saturation)
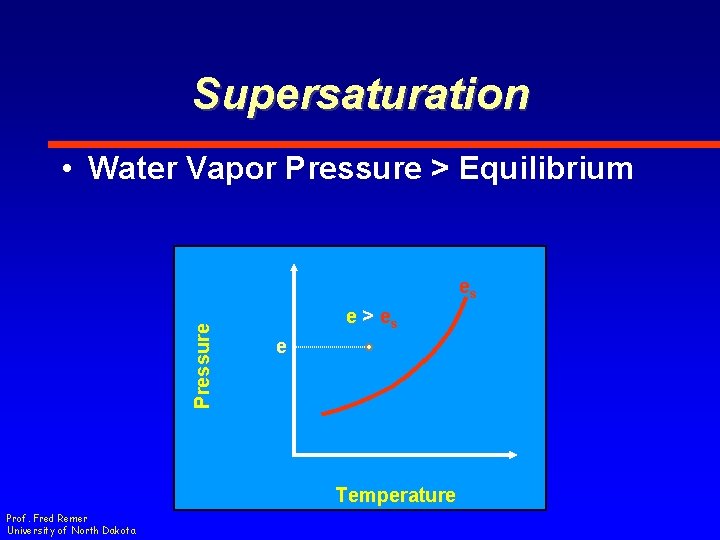
Supersaturation • Water Vapor Pressure > Equilibrium Pressure es e > es e Temperature Prof. Fred Remer University of North Dakota
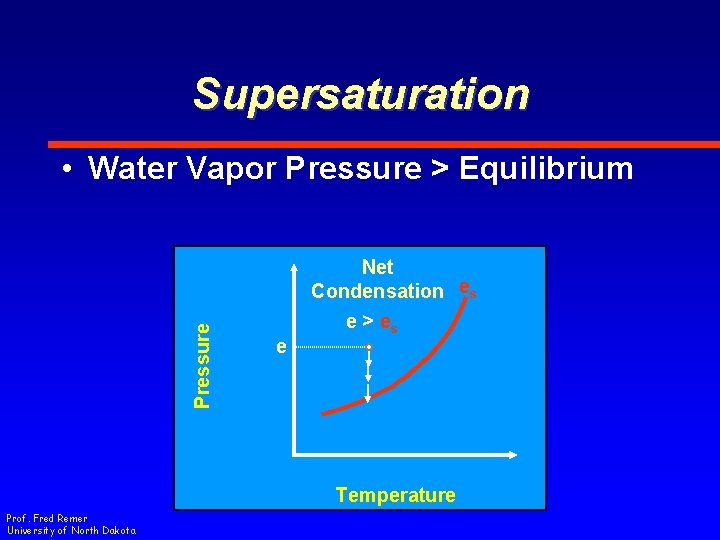
Supersaturation Pressure • Water Vapor Pressure > Equilibrium e Net Condensation es e > es Temperature Prof. Fred Remer University of North Dakota
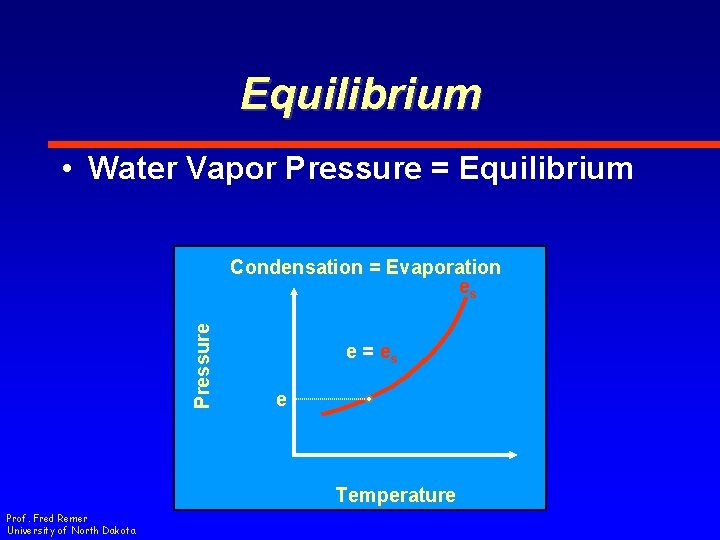
Equilibrium • Water Vapor Pressure = Equilibrium Pressure Condensation = Evaporation es e = es e Temperature Prof. Fred Remer University of North Dakota
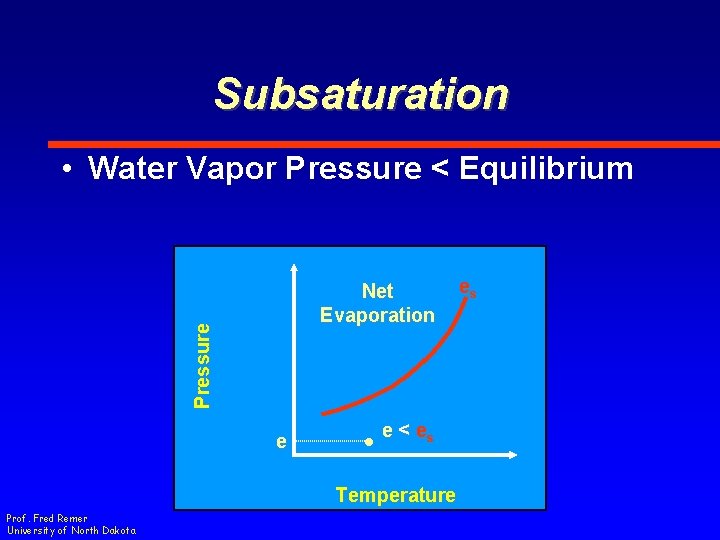
Subsaturation • Water Vapor Pressure < Equilibrium Pressure Net Evaporation e e < es Temperature Prof. Fred Remer University of North Dakota es
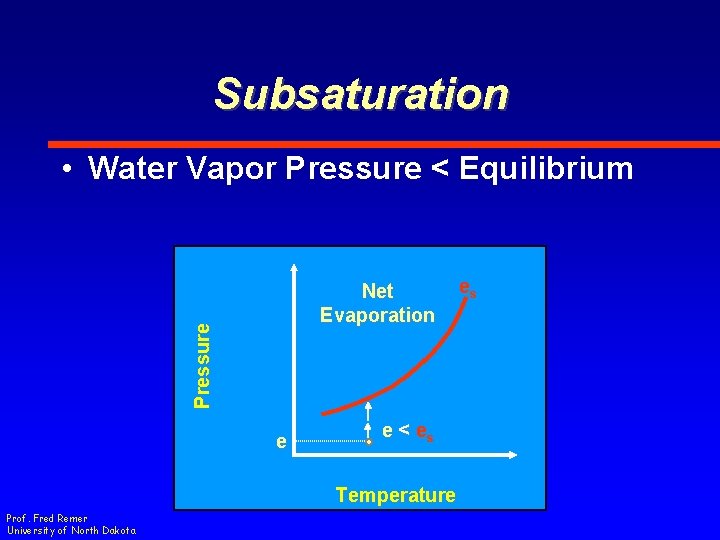
Subsaturation • Water Vapor Pressure < Equilibrium Pressure Net Evaporation e e < es Temperature Prof. Fred Remer University of North Dakota es
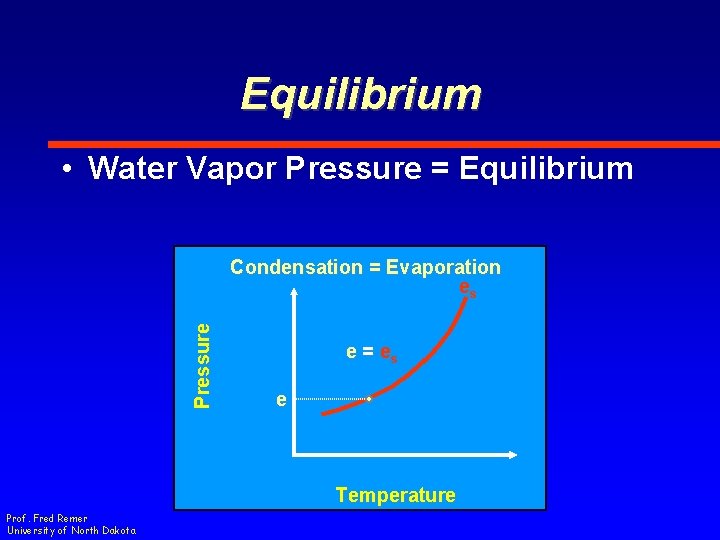
Equilibrium • Water Vapor Pressure = Equilibrium Pressure Condensation = Evaporation es e = es e Temperature Prof. Fred Remer University of North Dakota
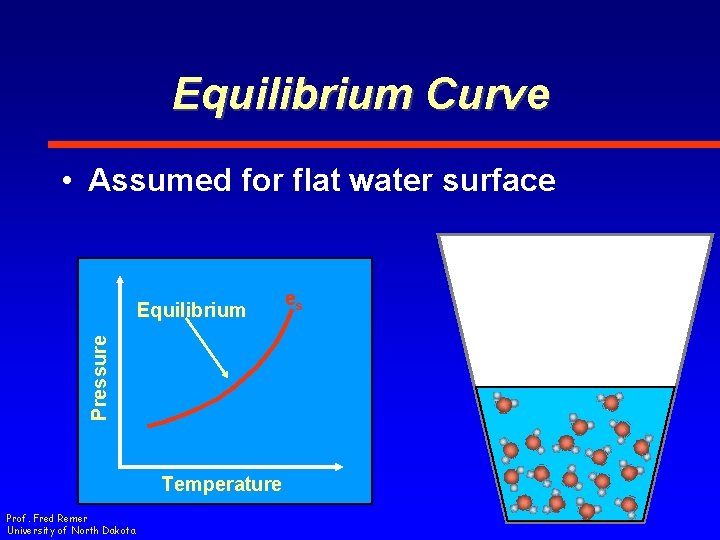
Equilibrium Curve • Assumed for flat water surface Pressure Equilibrium Temperature Prof. Fred Remer University of North Dakota es
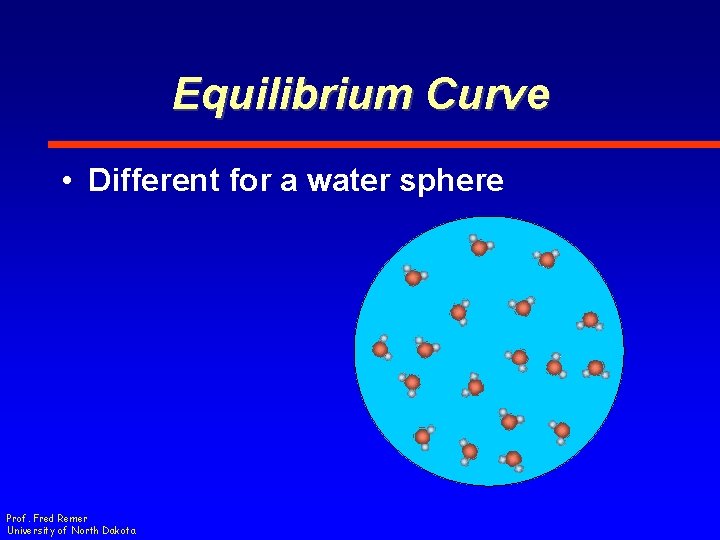
Equilibrium Curve • Different for a water sphere Prof. Fred Remer University of North Dakota
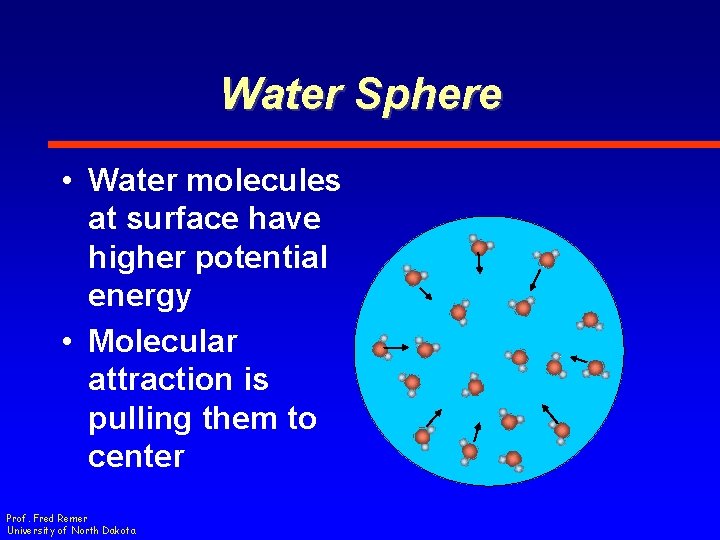
Water Sphere • Water molecules at surface have higher potential energy • Molecular attraction is pulling them to center Prof. Fred Remer University of North Dakota
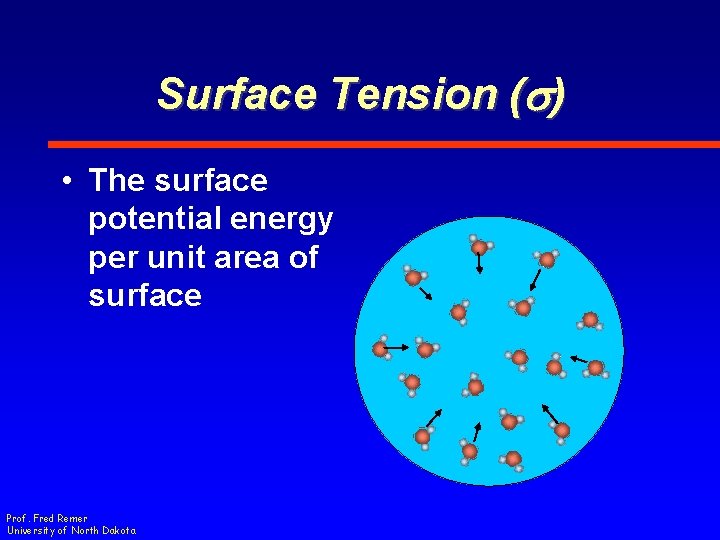
Surface Tension (s) • The surface potential energy per unit area of surface Prof. Fred Remer University of North Dakota
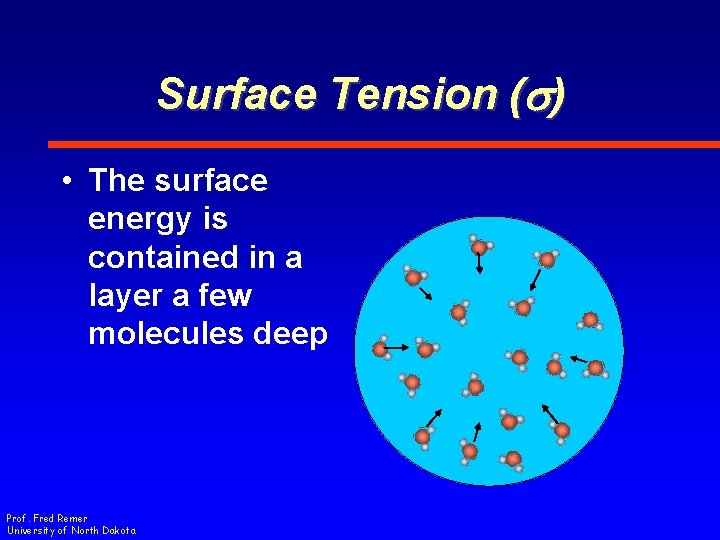
Surface Tension (s) • The surface energy is contained in a layer a few molecules deep Prof. Fred Remer University of North Dakota
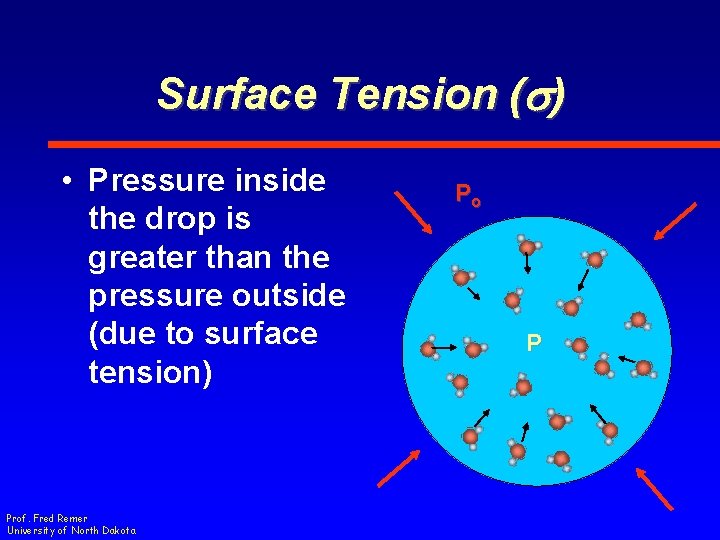
Surface Tension (s) • Pressure inside the drop is greater than the pressure outside (due to surface tension) Prof. Fred Remer University of North Dakota Po P
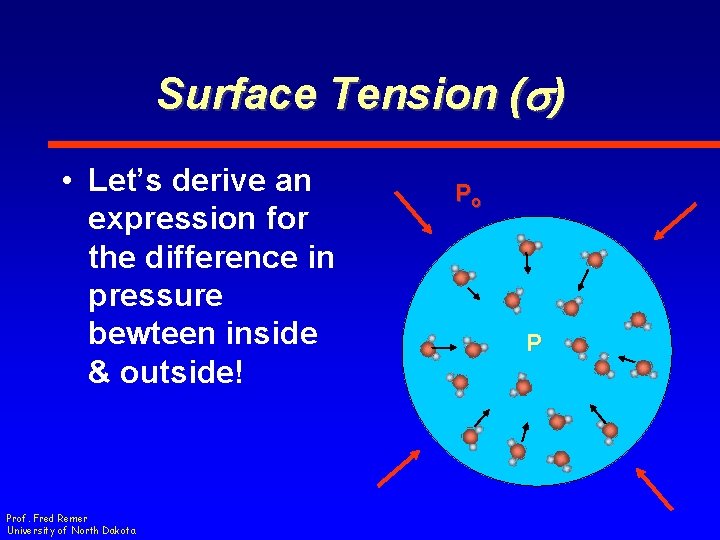
Surface Tension (s) • Let’s derive an expression for the difference in pressure bewteen inside & outside! Prof. Fred Remer University of North Dakota Po P
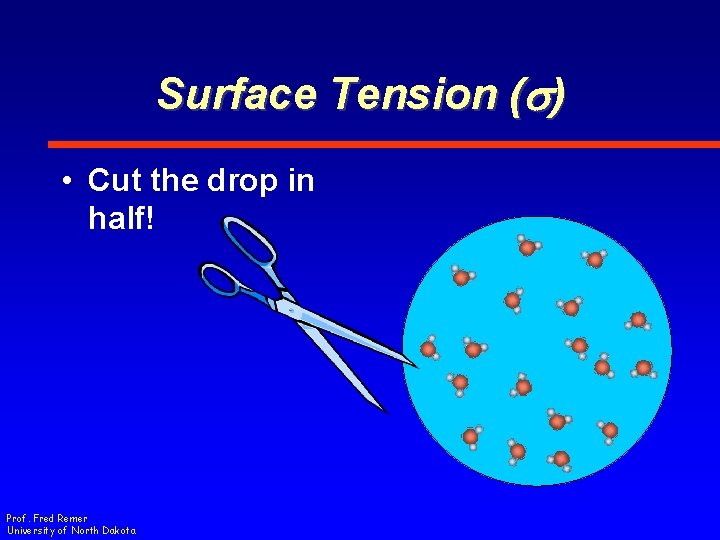
Surface Tension (s) • Cut the drop in half! Prof. Fred Remer University of North Dakota
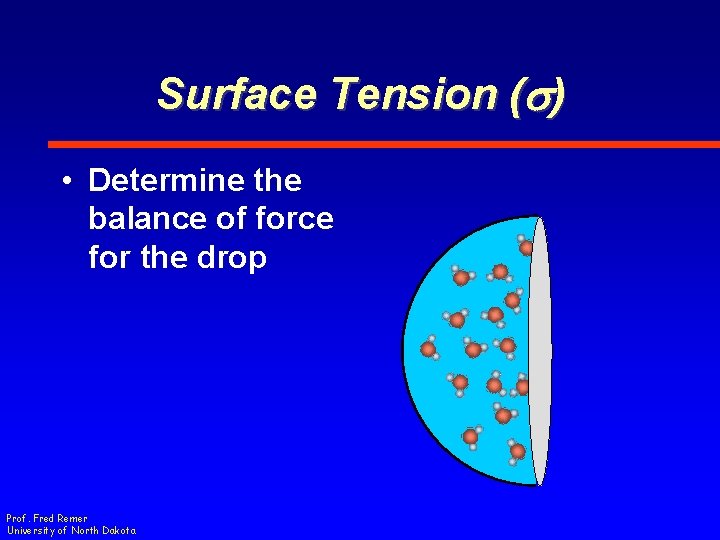
Surface Tension (s) • Determine the balance of force for the drop Prof. Fred Remer University of North Dakota
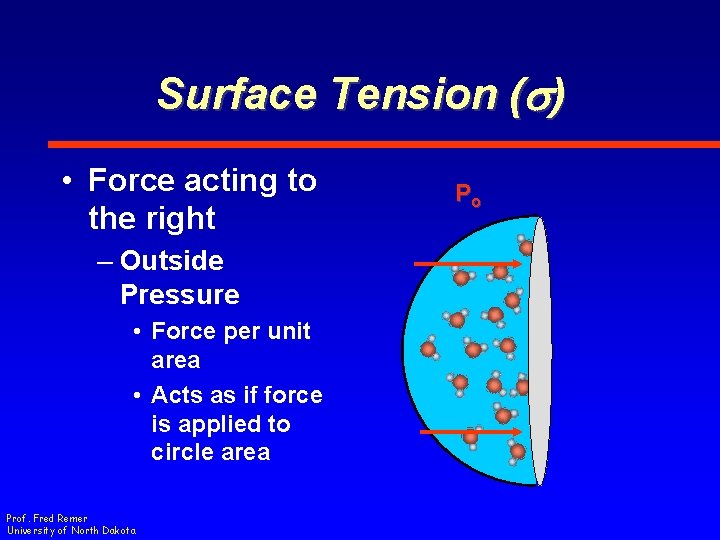
Surface Tension (s) • Force acting to the right – Outside Pressure • Force per unit area • Acts as if force is applied to circle area Prof. Fred Remer University of North Dakota Po
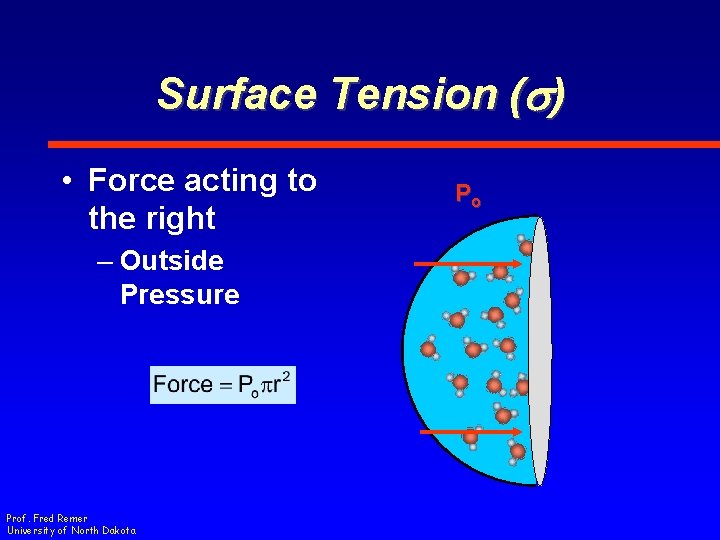
Surface Tension (s) • Force acting to the right – Outside Pressure Prof. Fred Remer University of North Dakota Po
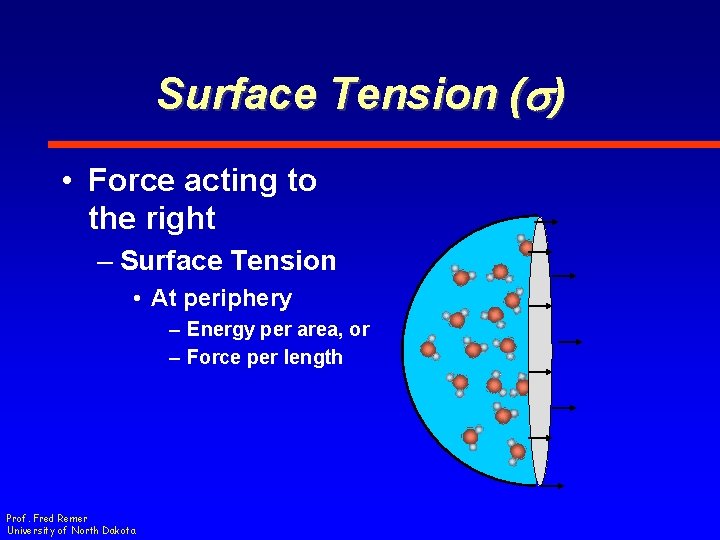
Surface Tension (s) • Force acting to the right – Surface Tension • At periphery – Energy per area, or – Force per length Prof. Fred Remer University of North Dakota
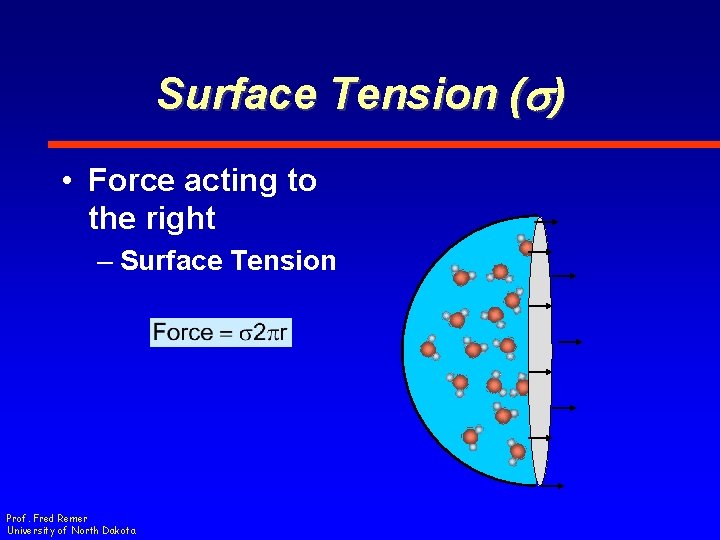
Surface Tension (s) • Force acting to the right – Surface Tension Prof. Fred Remer University of North Dakota
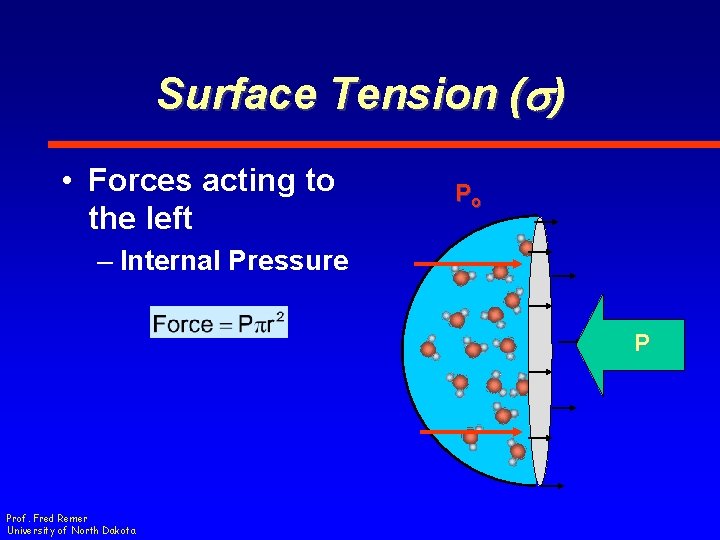
Surface Tension (s) • Forces acting to the left Po – Internal Pressure P Prof. Fred Remer University of North Dakota
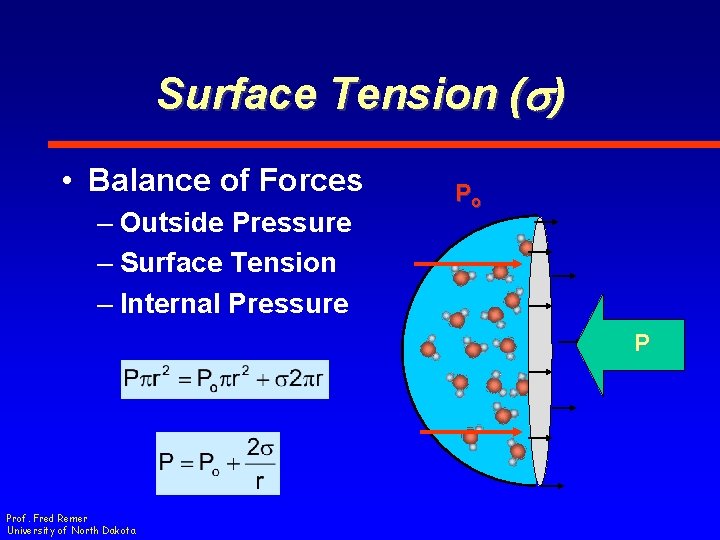
Surface Tension (s) • Balance of Forces – Outside Pressure – Surface Tension – Internal Pressure Po P Prof. Fred Remer University of North Dakota
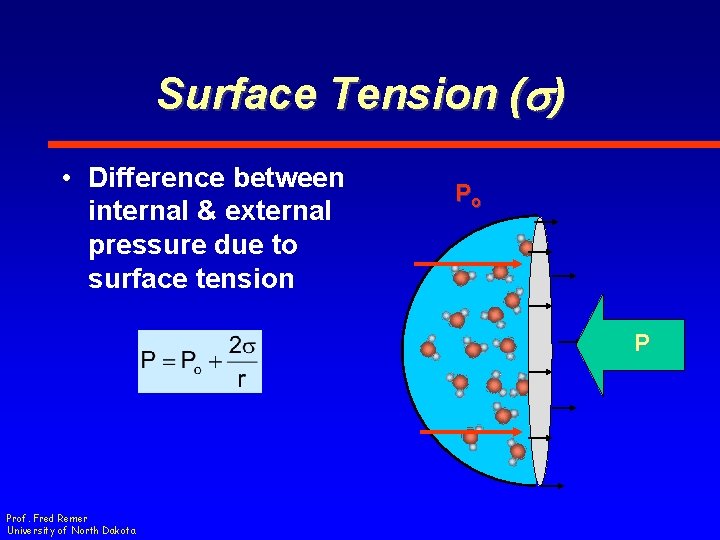
Surface Tension (s) • Difference between internal & external pressure due to surface tension Po P Prof. Fred Remer University of North Dakota
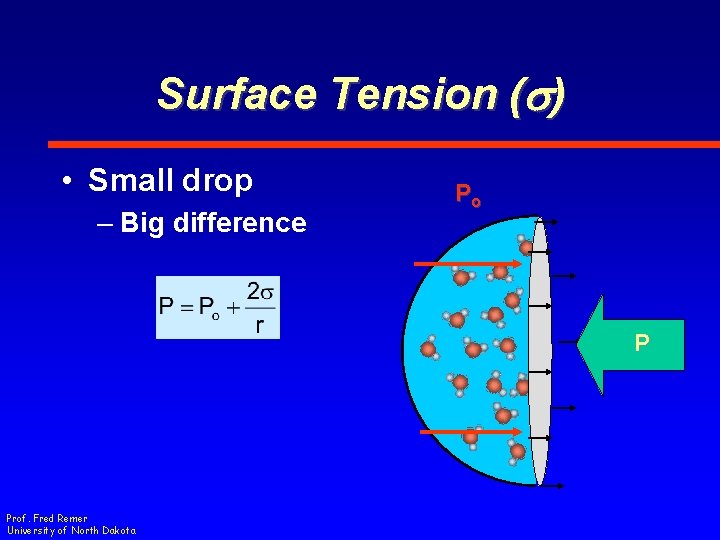
Surface Tension (s) • Small drop – Big difference Po P Prof. Fred Remer University of North Dakota
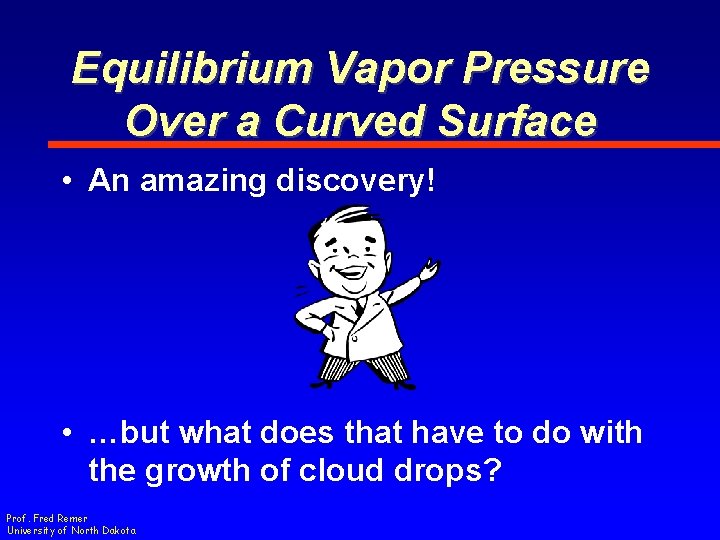
Equilibrium Vapor Pressure Over a Curved Surface • An amazing discovery! • …but what does that have to do with the growth of cloud drops? Prof. Fred Remer University of North Dakota
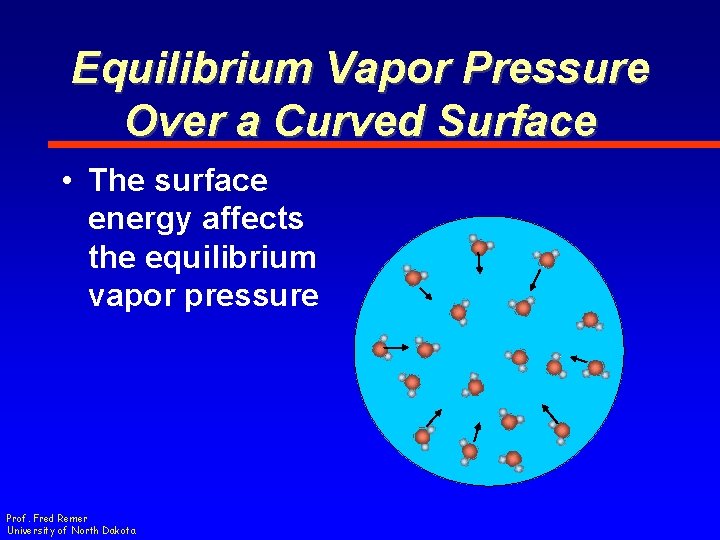
Equilibrium Vapor Pressure Over a Curved Surface • The surface energy affects the equilibrium vapor pressure Prof. Fred Remer University of North Dakota
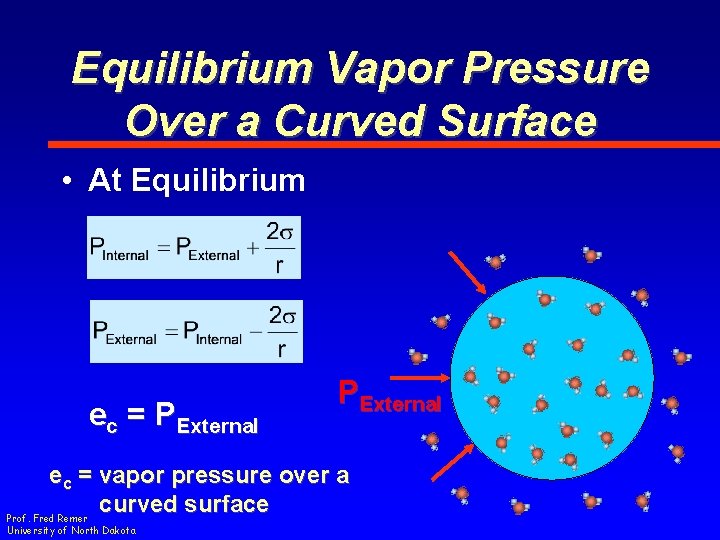
Equilibrium Vapor Pressure Over a Curved Surface • At Equilibrium ec = PExternal ec = vapor pressure over a curved surface Prof. Fred Remer University of North Dakota
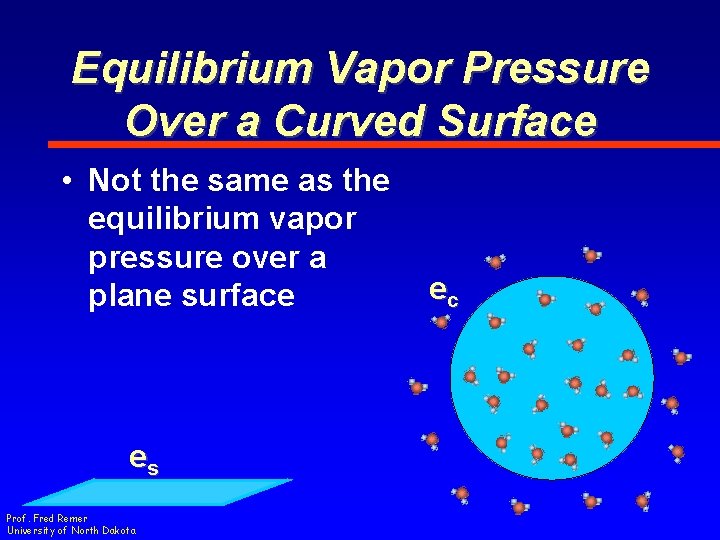
Equilibrium Vapor Pressure Over a Curved Surface • Not the same as the equilibrium vapor pressure over a plane surface es Prof. Fred Remer University of North Dakota ec
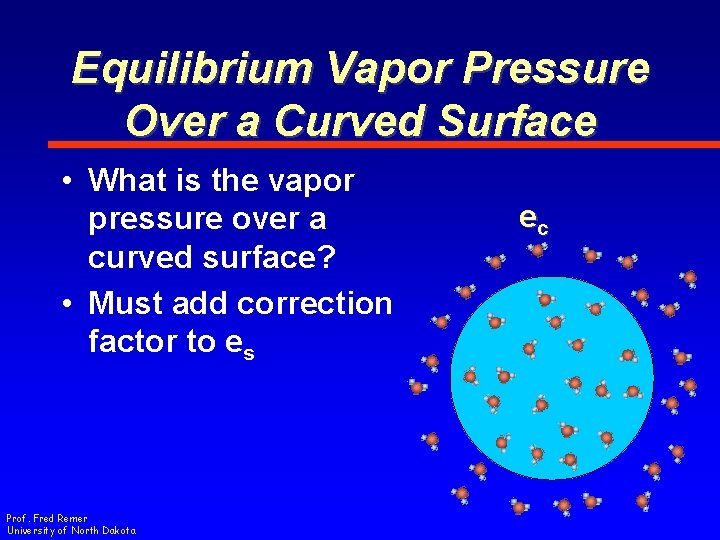
Equilibrium Vapor Pressure Over a Curved Surface • What is the vapor pressure over a curved surface? • Must add correction factor to es Prof. Fred Remer University of North Dakota ec
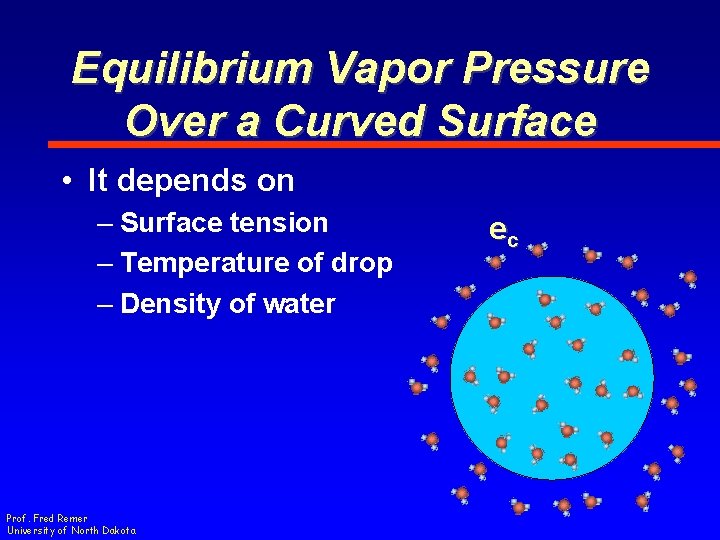
Equilibrium Vapor Pressure Over a Curved Surface • It depends on – Surface tension – Temperature of drop – Density of water Prof. Fred Remer University of North Dakota ec
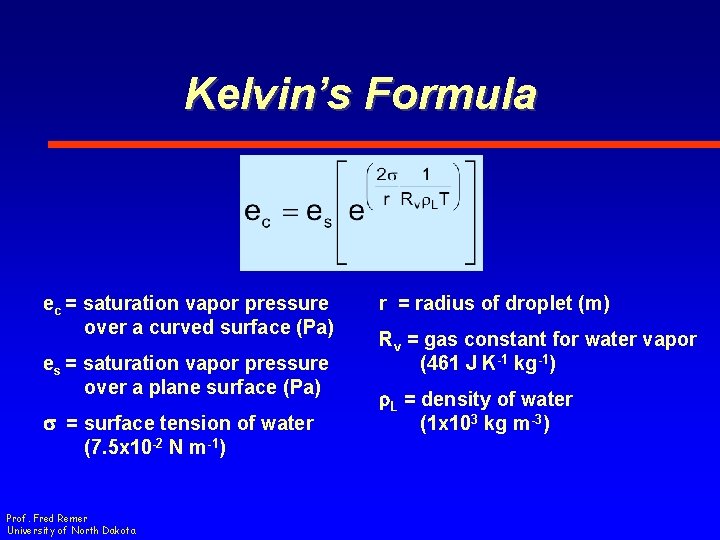
Kelvin’s Formula ec = saturation vapor pressure over a curved surface (Pa) es = saturation vapor pressure over a plane surface (Pa) s = surface tension of water (7. 5 x 10 -2 N m-1) Prof. Fred Remer University of North Dakota r = radius of droplet (m) Rv = gas constant for water vapor (461 J K-1 kg-1) r. L = density of water (1 x 103 kg m-3)
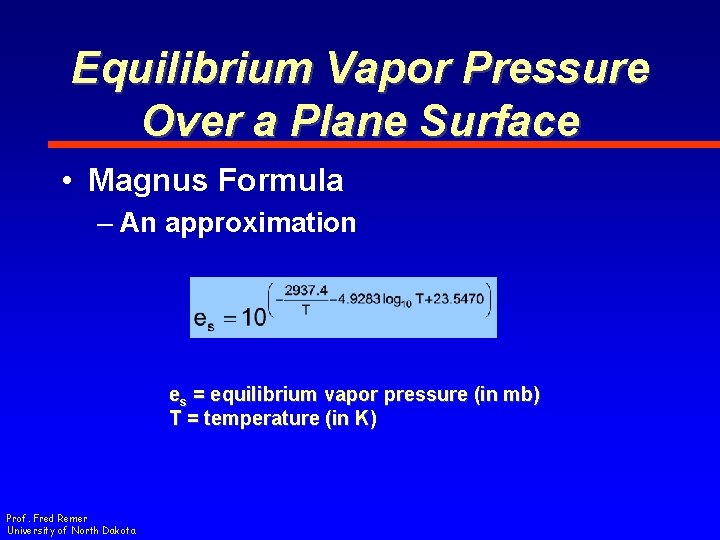
Equilibrium Vapor Pressure Over a Plane Surface • Magnus Formula – An approximation es = equilibrium vapor pressure (in mb) T = temperature (in K) Prof. Fred Remer University of North Dakota
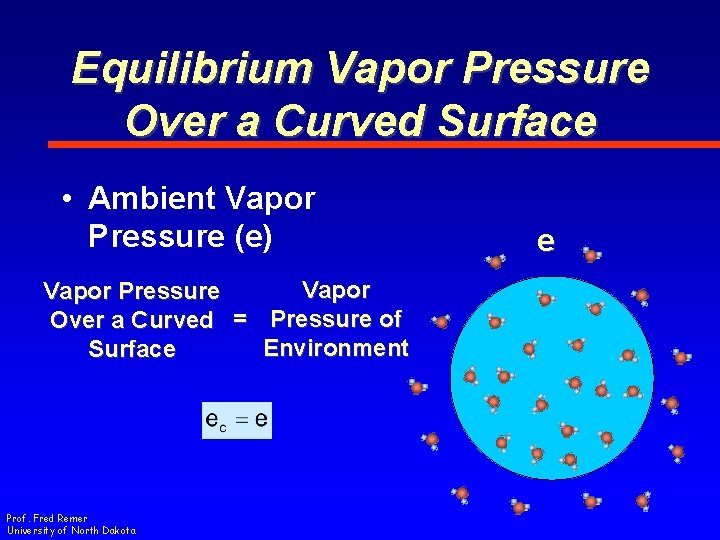
Equilibrium Vapor Pressure Over a Curved Surface • Ambient Vapor Pressure (e) Vapor Pressure Over a Curved = Pressure of Environment Surface Prof. Fred Remer University of North Dakota e
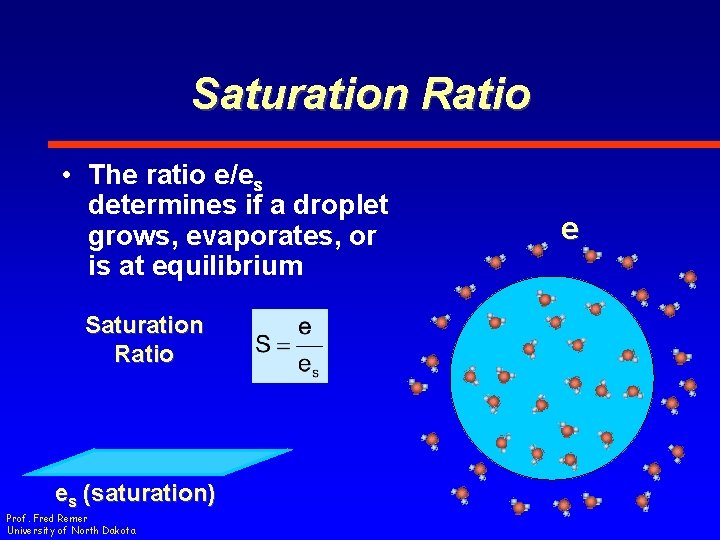
Saturation Ratio • The ratio e/es determines if a droplet grows, evaporates, or is at equilibrium Saturation Ratio es (saturation) Prof. Fred Remer University of North Dakota e
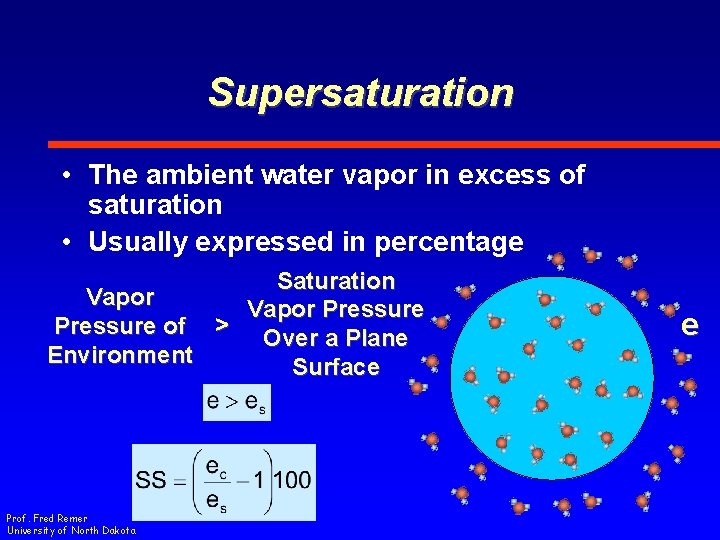
Supersaturation • The ambient water vapor in excess of saturation • Usually expressed in percentage Saturation Vapor Pressure of > Over a Plane Environment Surface Prof. Fred Remer University of North Dakota e
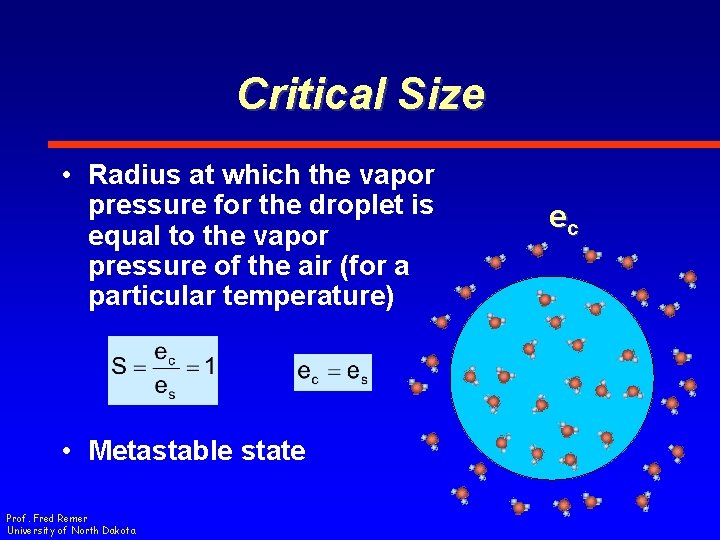
Critical Size • Radius at which the vapor pressure for the droplet is equal to the vapor pressure of the air (for a particular temperature) • Metastable state Prof. Fred Remer University of North Dakota ec
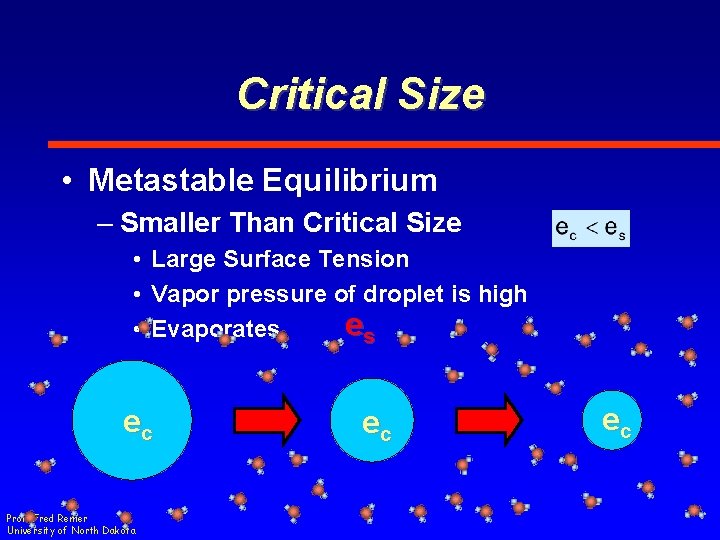
Critical Size • Metastable Equilibrium – Smaller Than Critical Size • Large Surface Tension • Vapor pressure of droplet is high es • Evaporates ec Prof. Fred Remer University of North Dakota ec ec
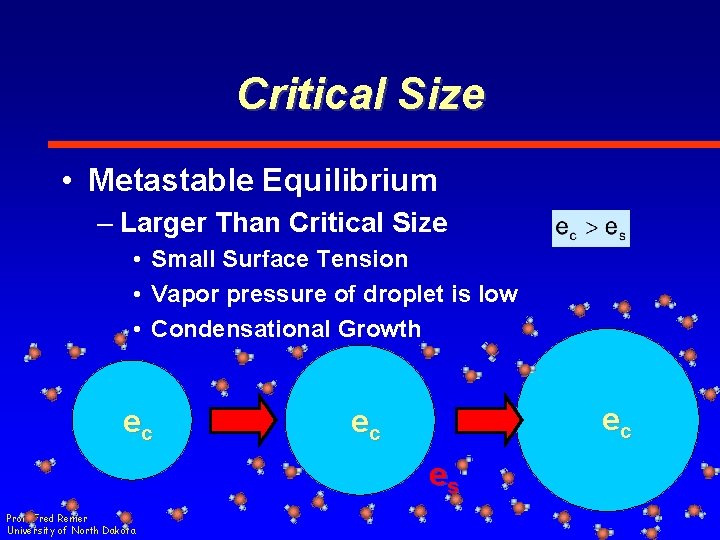
Critical Size • Metastable Equilibrium – Larger Than Critical Size • Small Surface Tension • Vapor pressure of droplet is low • Condensational Growth ec ec ec es Prof. Fred Remer University of North Dakota
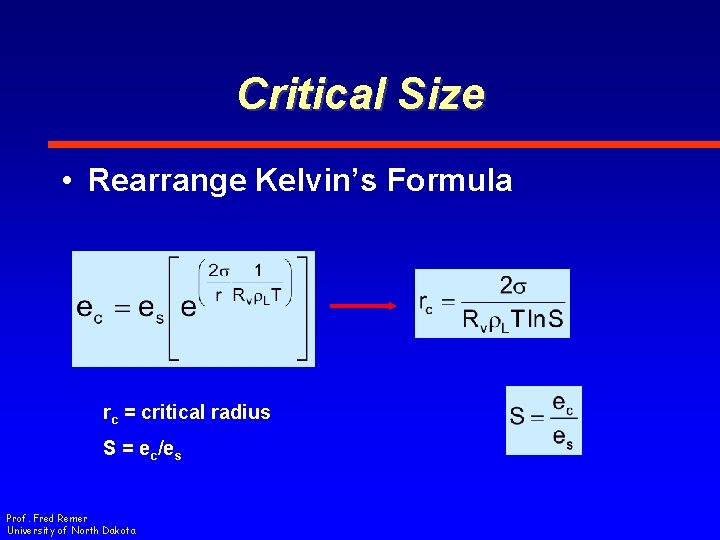
Critical Size • Rearrange Kelvin’s Formula rc = critical radius S = ec/es Prof. Fred Remer University of North Dakota
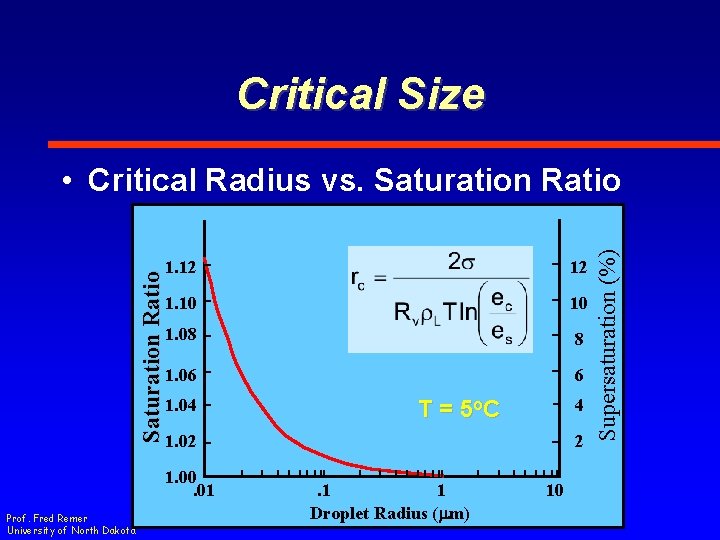
Critical Size 1. 12 12 1. 10 10 1. 08 8 1. 06 6 1. 04 1. 02 1. 00. 01 Prof. Fred Remer University of North Dakota 4 T = 5 o. C 2. 1 1 Droplet Radius (mm) 10 Supersaturation (%) Saturation Ratio • Critical Radius vs. Saturation Ratio
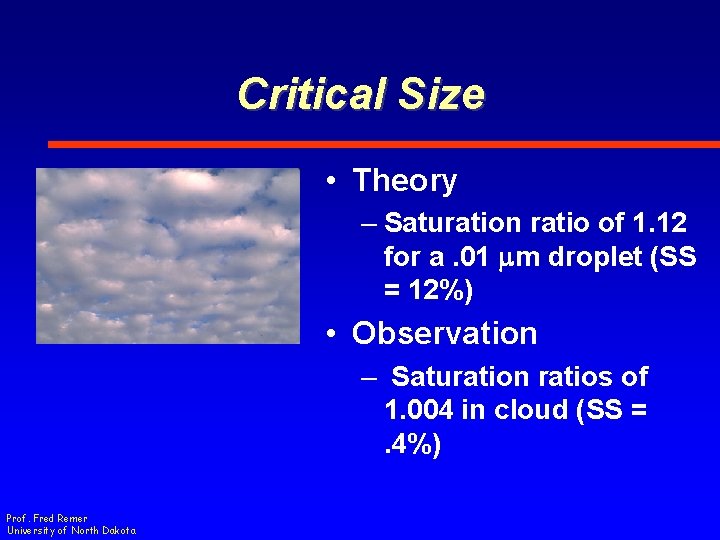
Critical Size • Theory – Saturation ratio of 1. 12 for a. 01 mm droplet (SS = 12%) • Observation – Saturation ratios of 1. 004 in cloud (SS =. 4%) Prof. Fred Remer University of North Dakota
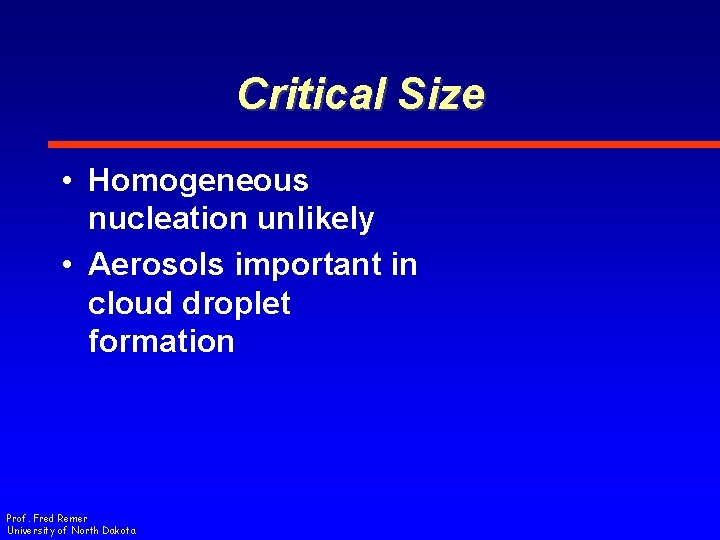
Critical Size • Homogeneous nucleation unlikely • Aerosols important in cloud droplet formation Prof. Fred Remer University of North Dakota
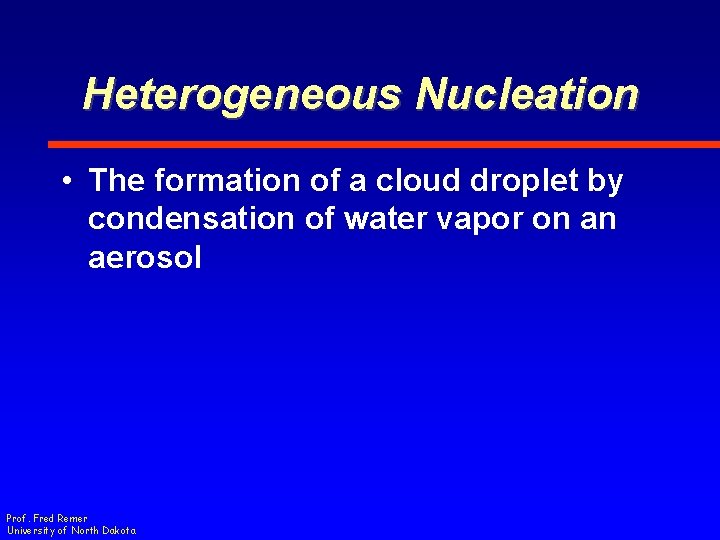
Heterogeneous Nucleation • The formation of a cloud droplet by condensation of water vapor on an aerosol Prof. Fred Remer University of North Dakota
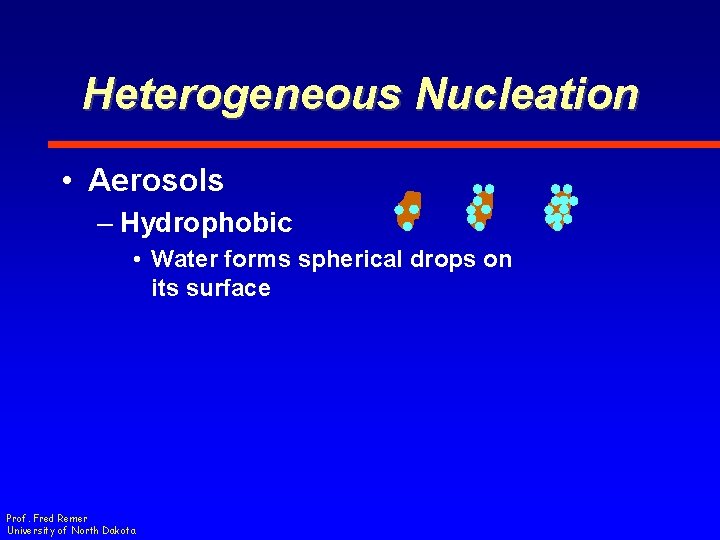
Heterogeneous Nucleation • Aerosols – Hydrophobic • Water forms spherical drops on its surface Prof. Fred Remer University of North Dakota
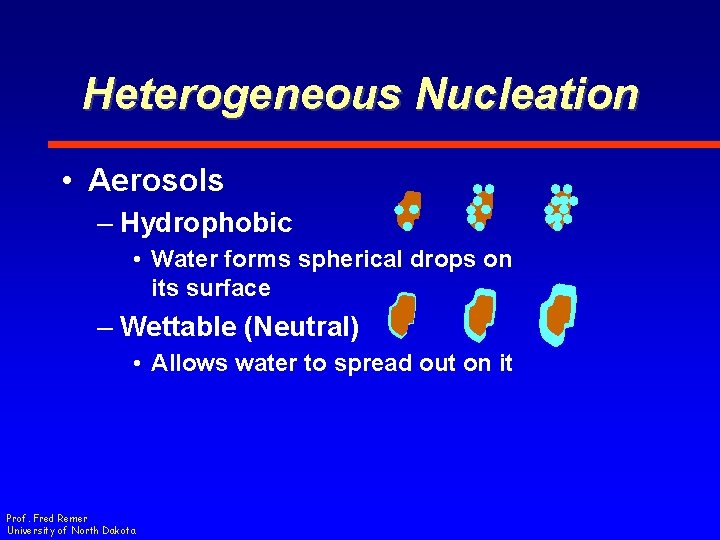
Heterogeneous Nucleation • Aerosols – Hydrophobic • Water forms spherical drops on its surface – Wettable (Neutral) • Allows water to spread out on it Prof. Fred Remer University of North Dakota
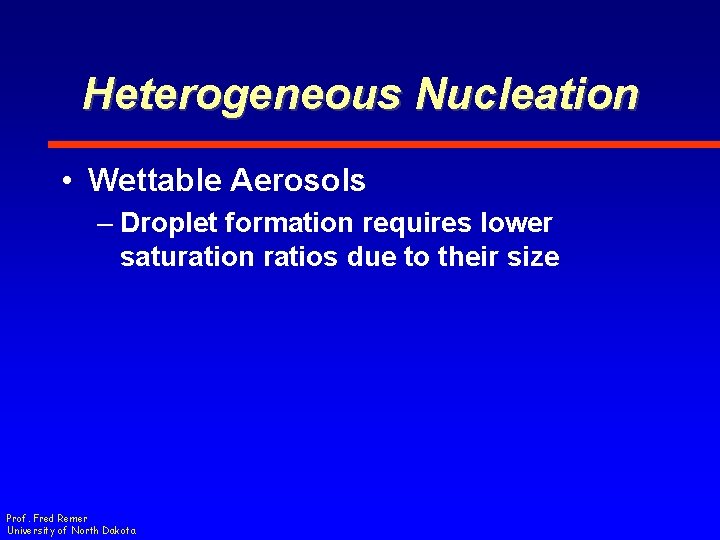
Heterogeneous Nucleation • Wettable Aerosols – Droplet formation requires lower saturation ratios due to their size Prof. Fred Remer University of North Dakota
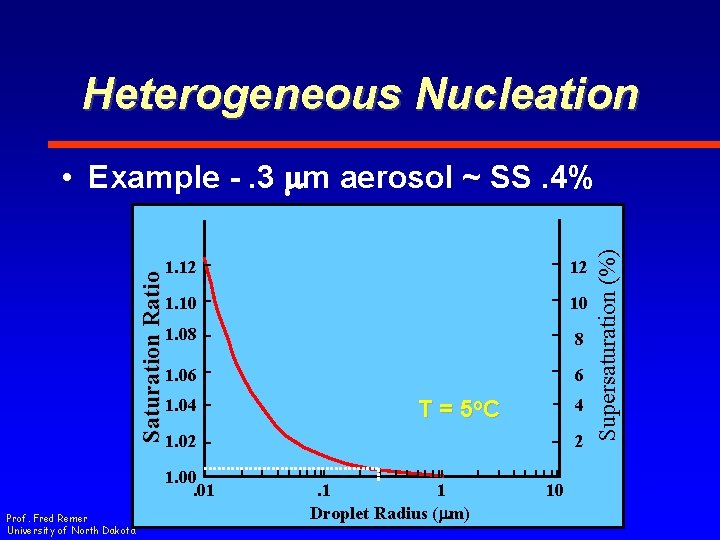
Heterogeneous Nucleation 1. 12 12 1. 10 10 1. 08 8 1. 06 6 1. 04 1. 02 1. 00. 01 Prof. Fred Remer University of North Dakota 4 T = 5 o. C 2. 1 1 Droplet Radius (mm) 10 Supersaturation (%) Saturation Ratio • Example -. 3 mm aerosol ~ SS. 4%
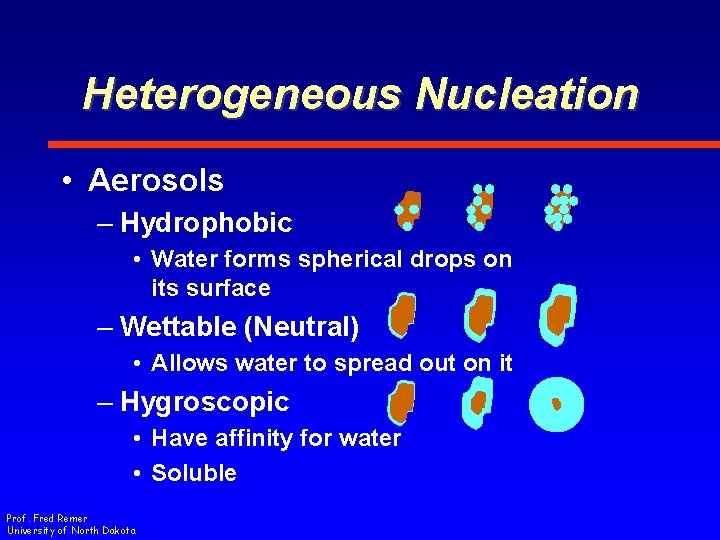
Heterogeneous Nucleation • Aerosols – Hydrophobic • Water forms spherical drops on its surface – Wettable (Neutral) • Allows water to spread out on it – Hygroscopic • Have affinity for water • Soluble Prof. Fred Remer University of North Dakota
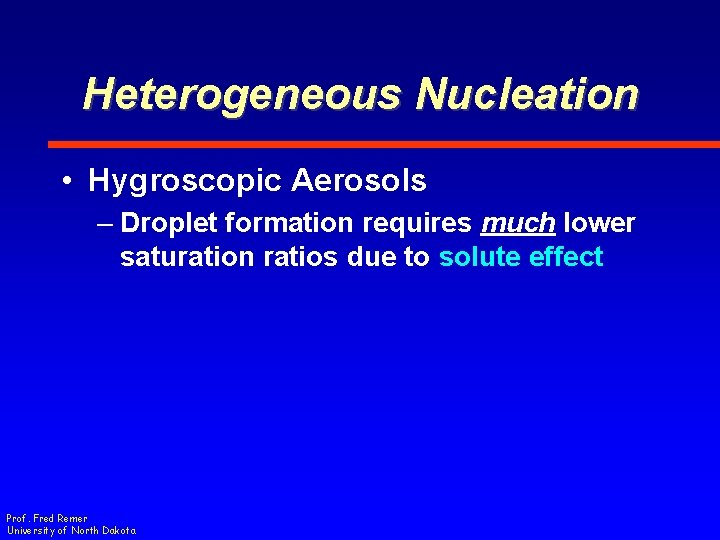
Heterogeneous Nucleation • Hygroscopic Aerosols – Droplet formation requires much lower saturation ratios due to solute effect Prof. Fred Remer University of North Dakota
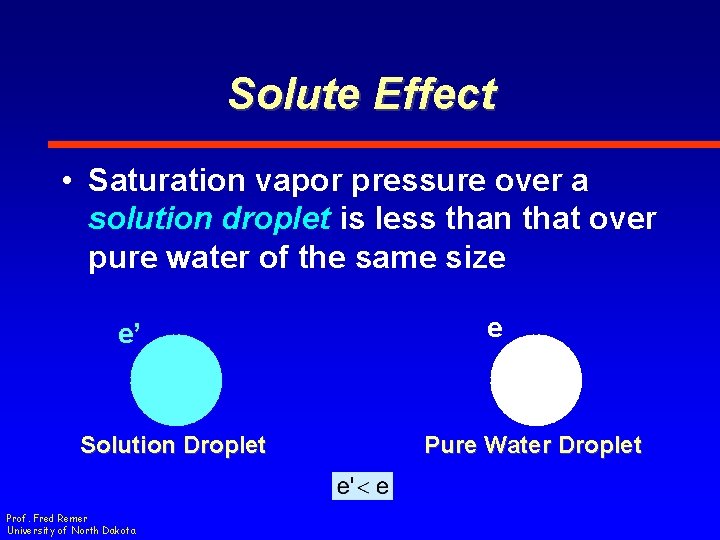
Solute Effect • Saturation vapor pressure over a solution droplet is less than that over pure water of the same size e’ Solution Droplet Prof. Fred Remer University of North Dakota e Pure Water Droplet
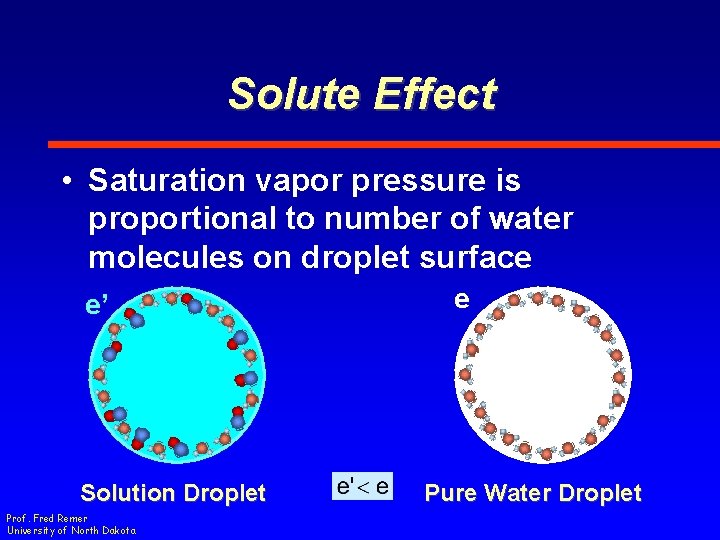
Solute Effect • Saturation vapor pressure is proportional to number of water molecules on droplet surface e’ e Solution Droplet Prof. Fred Remer University of North Dakota e e Pure Water Droplet
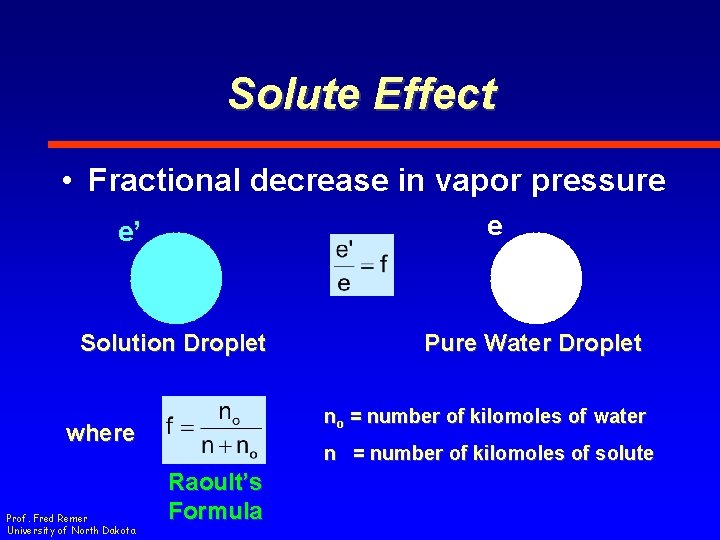
Solute Effect • Fractional decrease in vapor pressure e e’ Solution Droplet no = number of kilomoles of water where Prof. Fred Remer University of North Dakota Pure Water Droplet n = number of kilomoles of solute Raoult’s Formula
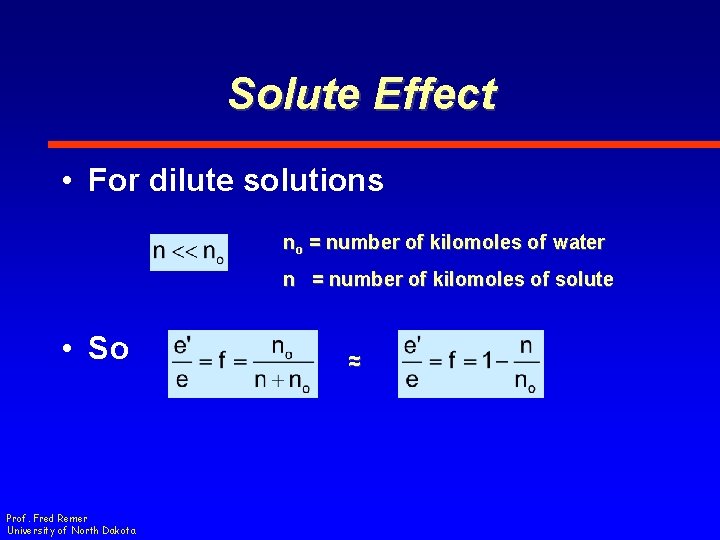
Solute Effect • For dilute solutions no = number of kilomoles of water n = number of kilomoles of solute • So Prof. Fred Remer University of North Dakota ~ ~
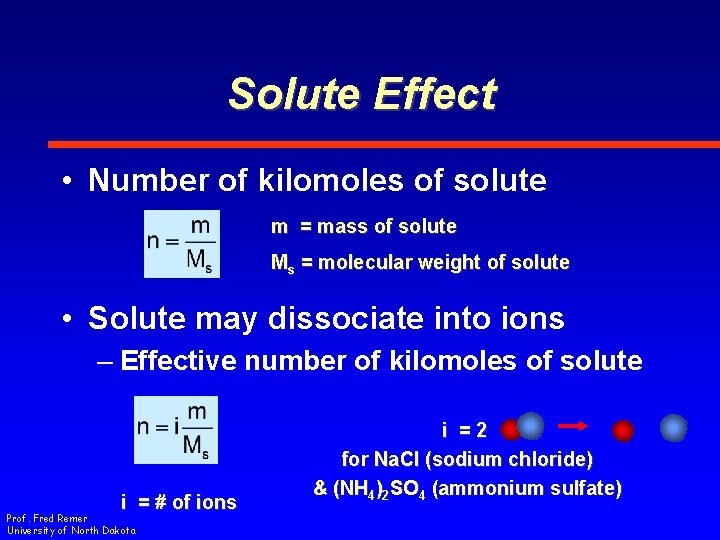
Solute Effect • Number of kilomoles of solute m = mass of solute Ms = molecular weight of solute • Solute may dissociate into ions – Effective number of kilomoles of solute i = # of ions Prof. Fred Remer University of North Dakota i =2 for Na. Cl (sodium chloride) & (NH 4)2 SO 4 (ammonium sulfate)
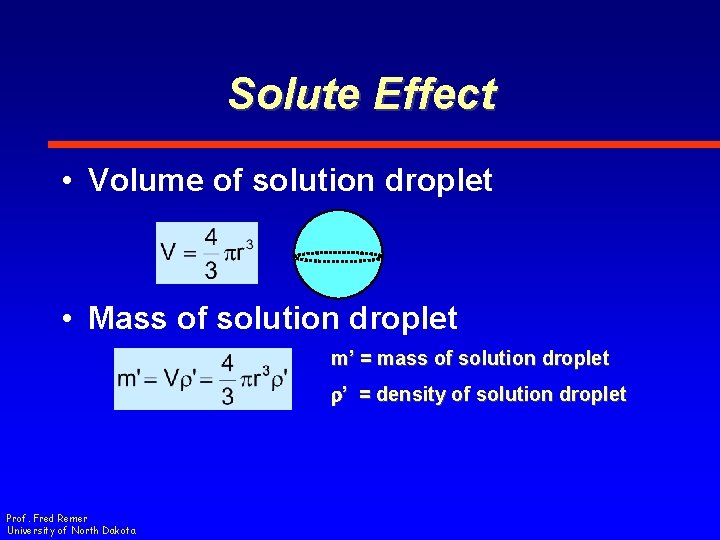
Solute Effect • Volume of solution droplet • Mass of solution droplet m’ = mass of solution droplet r’ = density of solution droplet Prof. Fred Remer University of North Dakota
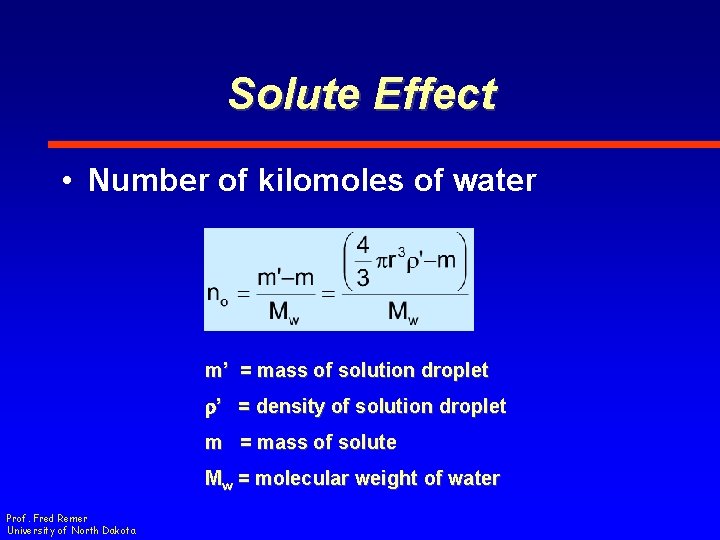
Solute Effect • Number of kilomoles of water m’ = mass of solution droplet r’ = density of solution droplet m = mass of solute Mw = molecular weight of water Prof. Fred Remer University of North Dakota
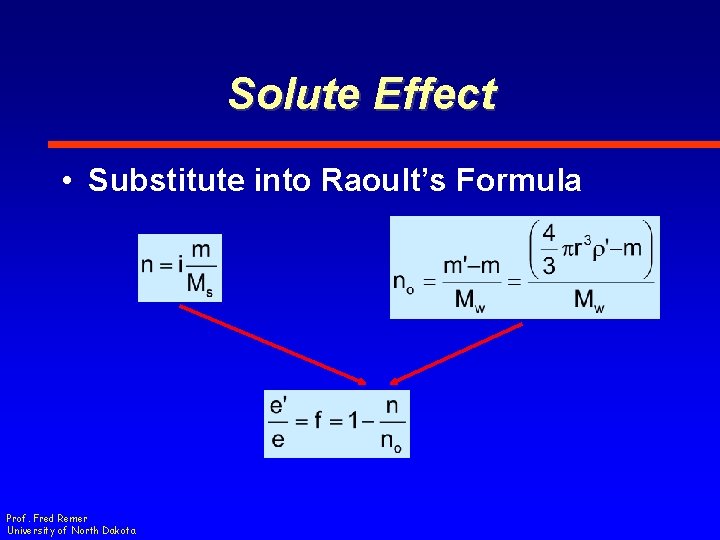
Solute Effect • Substitute into Raoult’s Formula Prof. Fred Remer University of North Dakota
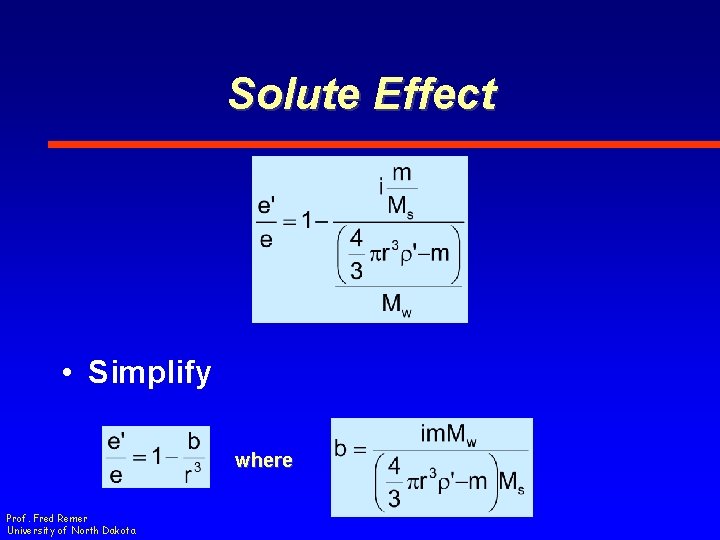
Solute Effect • Simplify where Prof. Fred Remer University of North Dakota
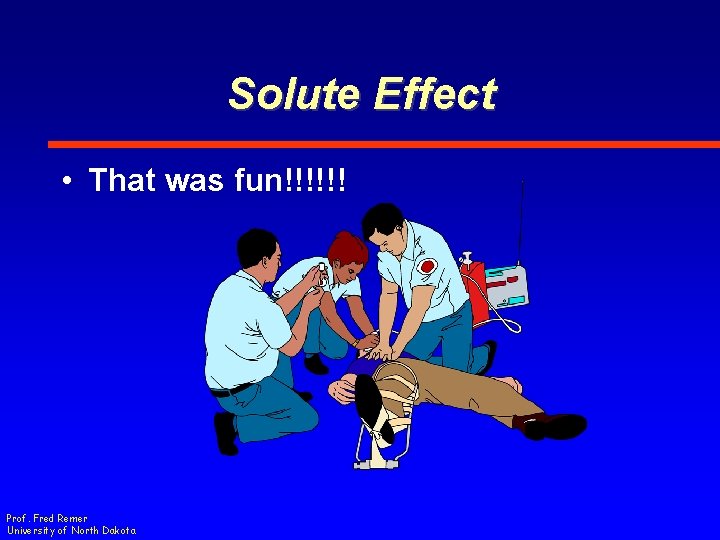
Solute Effect • That was fun!!!!!! Prof. Fred Remer University of North Dakota
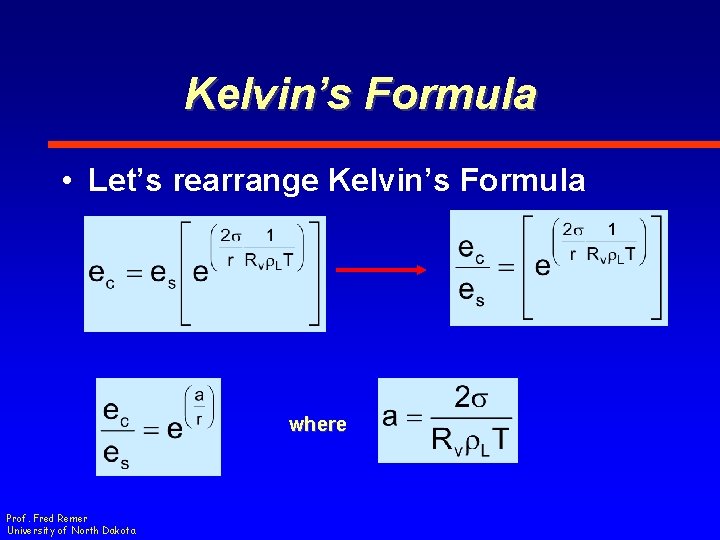
Kelvin’s Formula • Let’s rearrange Kelvin’s Formula where Prof. Fred Remer University of North Dakota
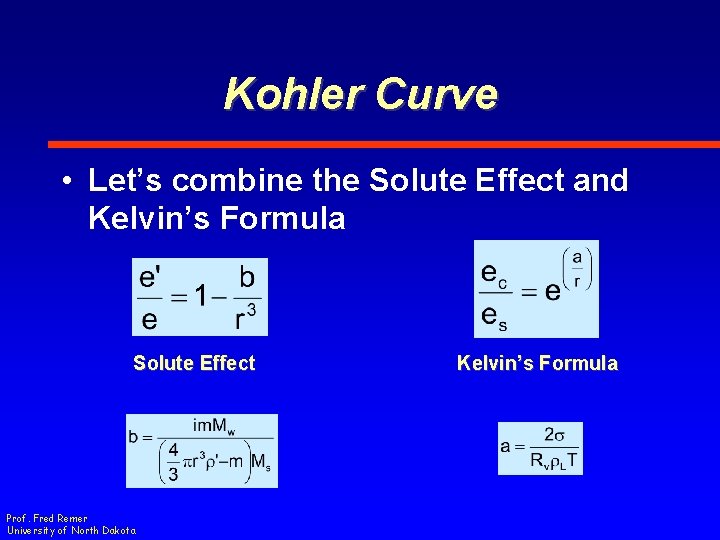
Kohler Curve • Let’s combine the Solute Effect and Kelvin’s Formula Solute Effect Prof. Fred Remer University of North Dakota Kelvin’s Formula
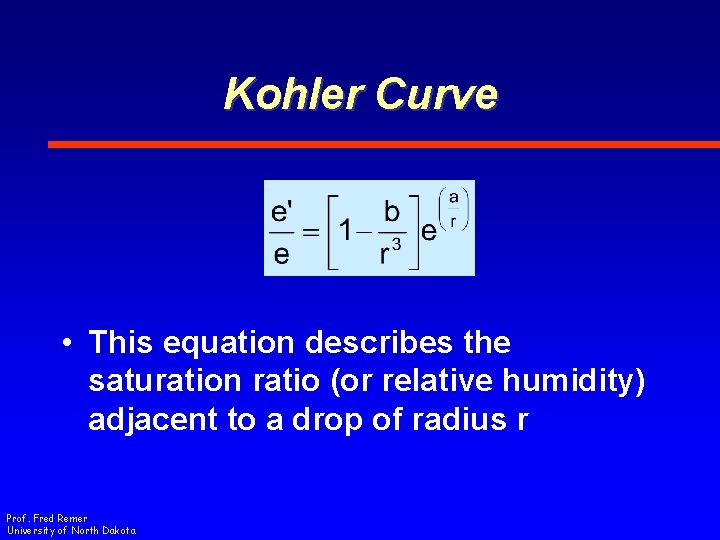
Kohler Curve • This equation describes the saturation ratio (or relative humidity) adjacent to a drop of radius r Prof. Fred Remer University of North Dakota
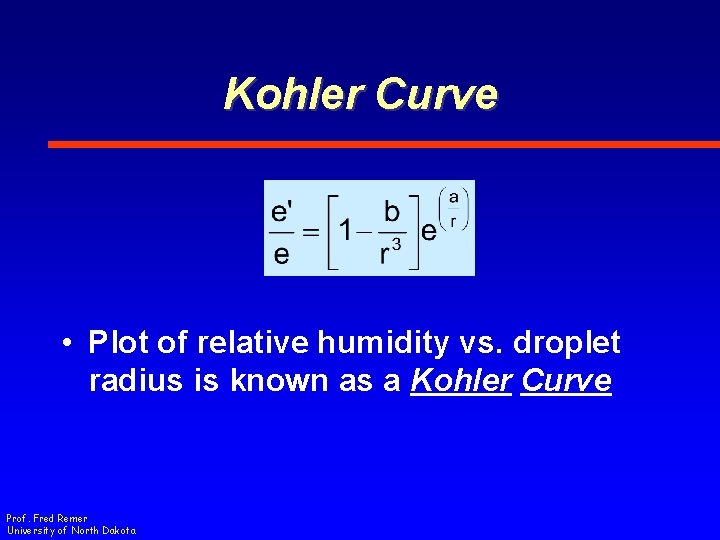
Kohler Curve • Plot of relative humidity vs. droplet radius is known as a Kohler Curve Prof. Fred Remer University of North Dakota
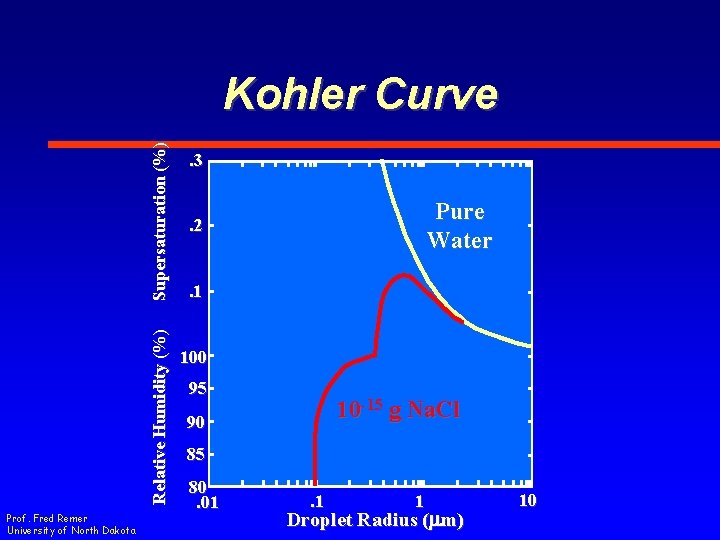
Relative Humidity (%) Supersaturation (%) Kohler Curve Prof. Fred Remer University of North Dakota . 3 Pure Water . 2 . 1 100 95 10 -15 g Na. Cl 90 85 80. 01 . 1 1 Droplet Radius (mm) 10
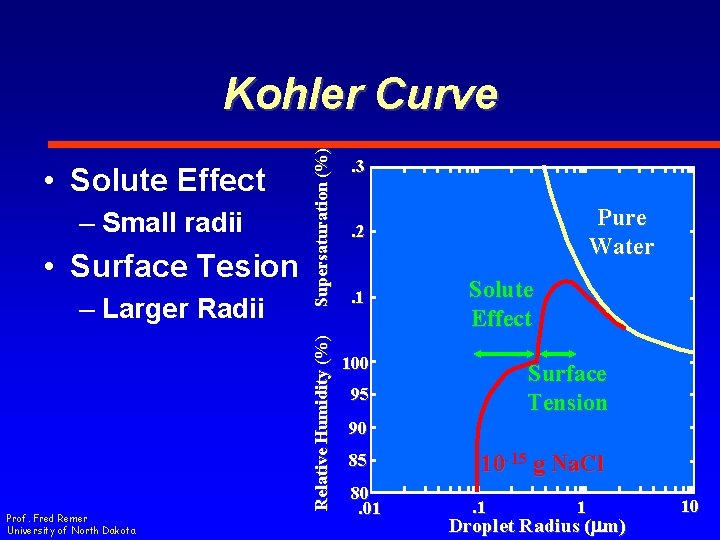
– Small radii • Surface Tesion – Larger Radii Prof. Fred Remer University of North Dakota Relative Humidity (%) • Solute Effect Supersaturation (%) Kohler Curve. 3 Pure Water . 2 . 1 Solute Effect 100 Surface Tension 95 90 85 80. 01 10 -15 g Na. Cl. 1 1 Droplet Radius (mm) 10
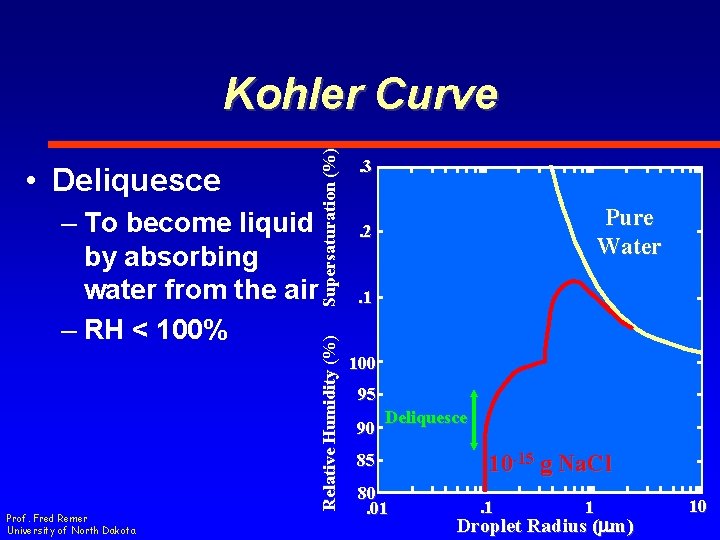
– To become liquid by absorbing water from the air – RH < 100% Prof. Fred Remer University of North Dakota Relative Humidity (%) • Deliquesce Supersaturation (%) Kohler Curve. 3 Pure Water . 2 . 1 100 95 90 Deliquesce 85 80. 01 10 -15 g Na. Cl. 1 1 Droplet Radius (mm) 10
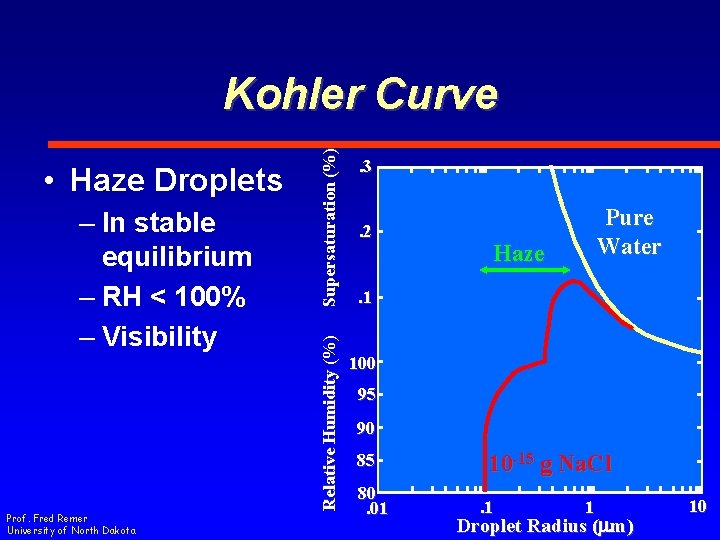
– In stable equilibrium – RH < 100% – Visibility Prof. Fred Remer University of North Dakota Relative Humidity (%) • Haze Droplets Supersaturation (%) Kohler Curve. 3. 2 Pure Water Haze . 1 100 95 90 85 80. 01 10 -15 g Na. Cl. 1 1 Droplet Radius (mm) 10
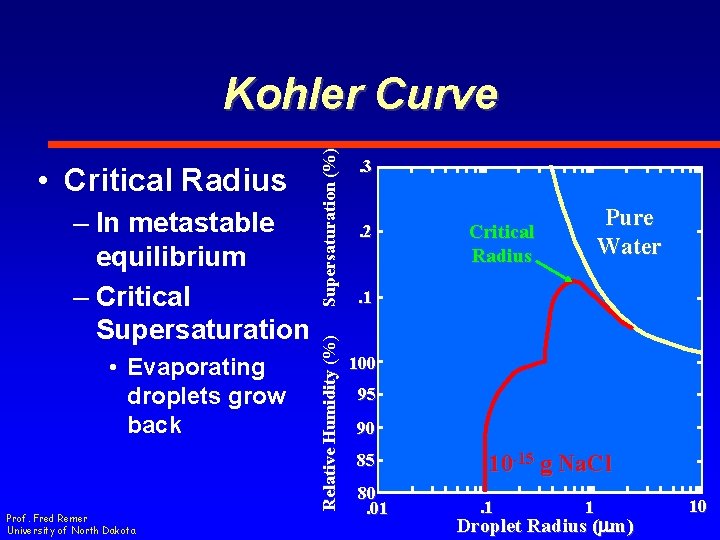
– In metastable equilibrium – Critical Supersaturation • Evaporating droplets grow back Prof. Fred Remer University of North Dakota Relative Humidity (%) • Critical Radius Supersaturation (%) Kohler Curve. 3. 2 Pure Water Critical Radius . 1 100 95 90 85 80. 01 10 -15 g Na. Cl. 1 1 Droplet Radius (mm) 10
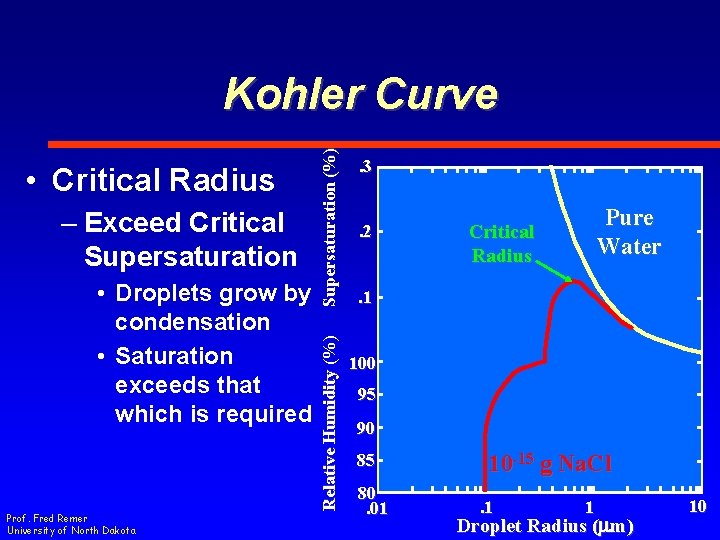
– Exceed Critical Supersaturation • Droplets grow by condensation • Saturation exceeds that which is required Prof. Fred Remer University of North Dakota Relative Humidity (%) • Critical Radius Supersaturation (%) Kohler Curve. 3. 2 Pure Water Critical Radius . 1 100 95 90 85 80. 01 10 -15 g Na. Cl. 1 1 Droplet Radius (mm) 10
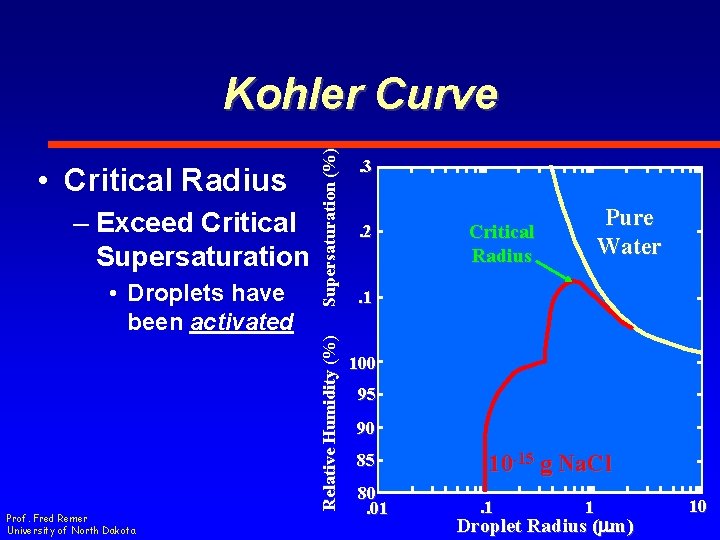
– Exceed Critical Supersaturation • Droplets have been activated Prof. Fred Remer University of North Dakota Relative Humidity (%) • Critical Radius Supersaturation (%) Kohler Curve. 3. 2 Pure Water Critical Radius . 1 100 95 90 85 80. 01 10 -15 g Na. Cl. 1 1 Droplet Radius (mm) 10
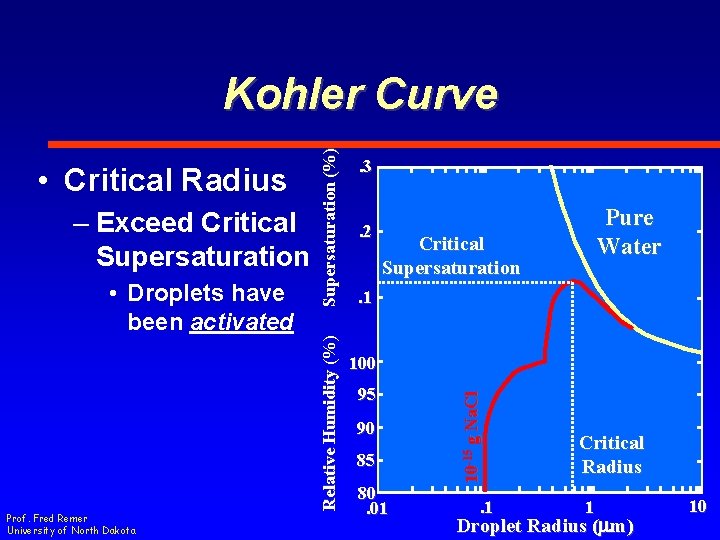
• Droplets have been activated Prof. Fred Remer University of North Dakota . 3. 2 Pure Water Critical Supersaturation . 1 100 95 90 85 80. 01 10 -15 g Na. Cl – Exceed Critical Supersaturation Relative Humidity (%) • Critical Radius Supersaturation (%) Kohler Curve . 1 Critical Radius 1 Droplet Radius (mm) 10
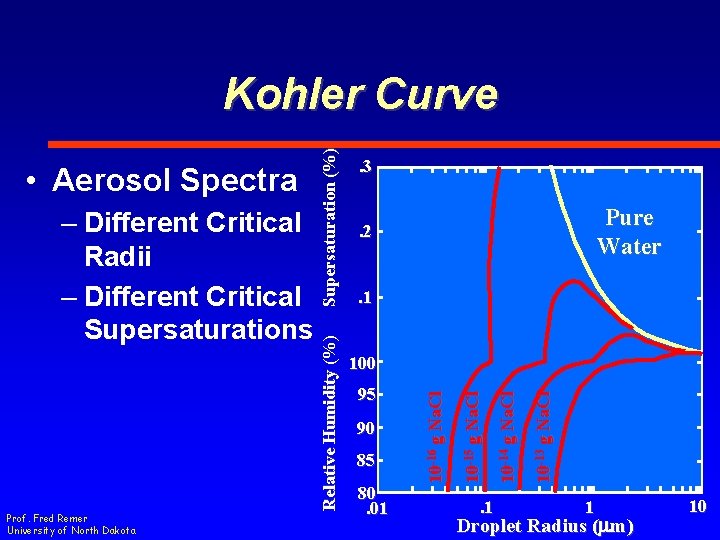
Prof. Fred Remer University of North Dakota . 3 Pure Water . 2 . 1 85 80. 01 . 1 10 -13 g Na. Cl 90 10 -14 g Na. Cl 95 10 -15 g Na. Cl 100 10 -16 g Na. Cl – Different Critical Radii – Different Critical Supersaturations Relative Humidity (%) • Aerosol Spectra Supersaturation (%) Kohler Curve 1 Droplet Radius (mm) 10
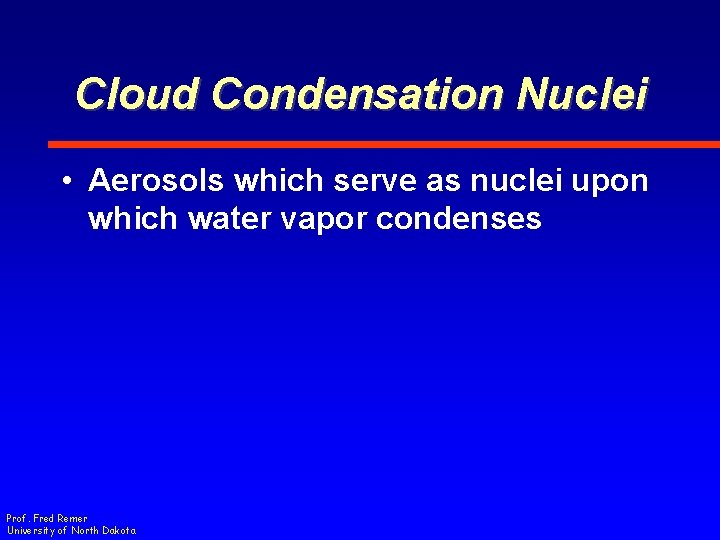
Cloud Condensation Nuclei • Aerosols which serve as nuclei upon which water vapor condenses Prof. Fred Remer University of North Dakota
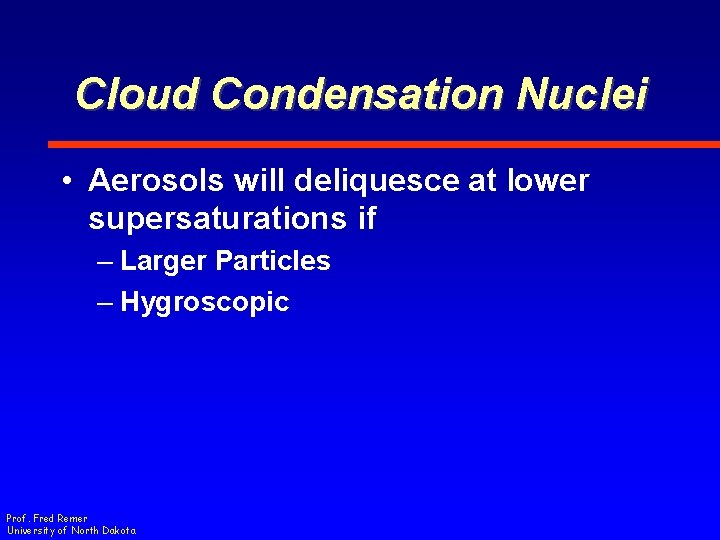
Cloud Condensation Nuclei • Aerosols will deliquesce at lower supersaturations if – Larger Particles – Hygroscopic Prof. Fred Remer University of North Dakota
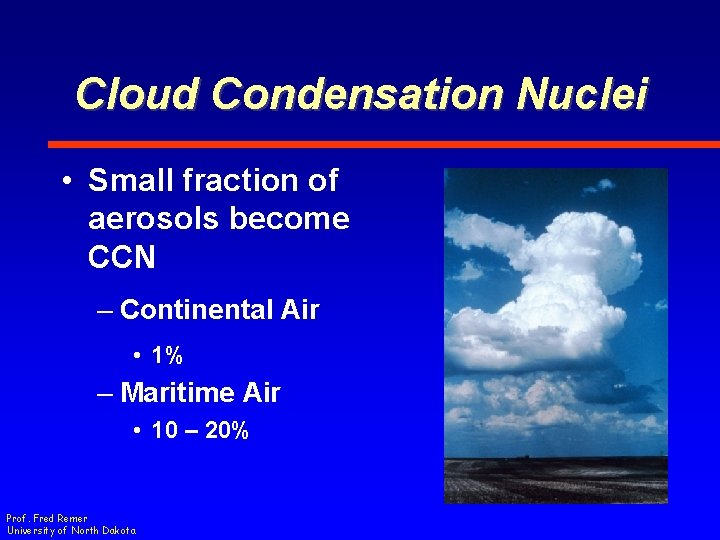
Cloud Condensation Nuclei • Small fraction of aerosols become CCN – Continental Air • 1% – Maritime Air • 10 – 20% Prof. Fred Remer University of North Dakota
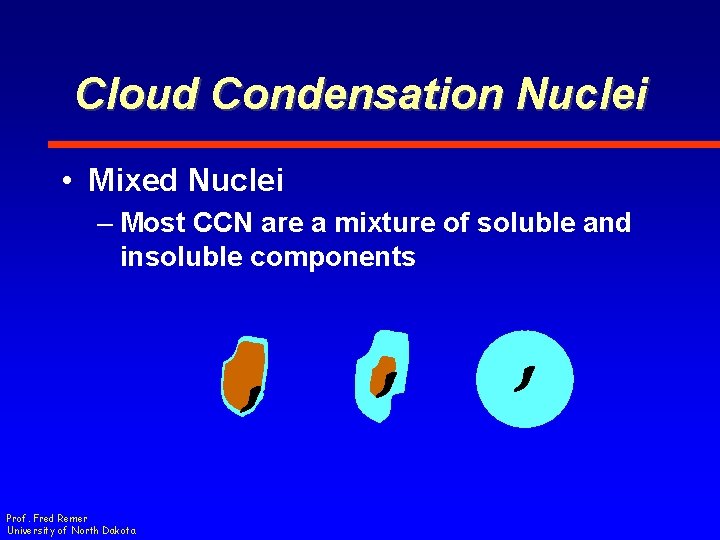
Cloud Condensation Nuclei • Mixed Nuclei – Most CCN are a mixture of soluble and insoluble components Prof. Fred Remer University of North Dakota
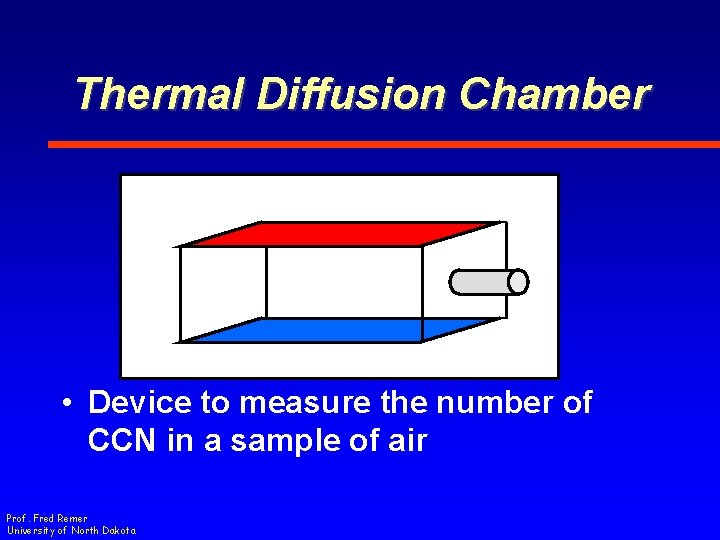
Thermal Diffusion Chamber • Device to measure the number of CCN in a sample of air Prof. Fred Remer University of North Dakota
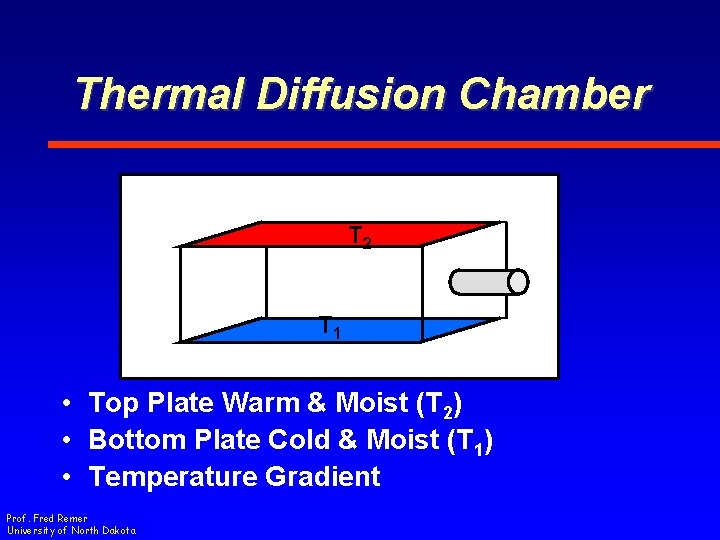
Thermal Diffusion Chamber T 2 T 1 • Top Plate Warm & Moist (T 2) • Bottom Plate Cold & Moist (T 1) • Temperature Gradient Prof. Fred Remer University of North Dakota
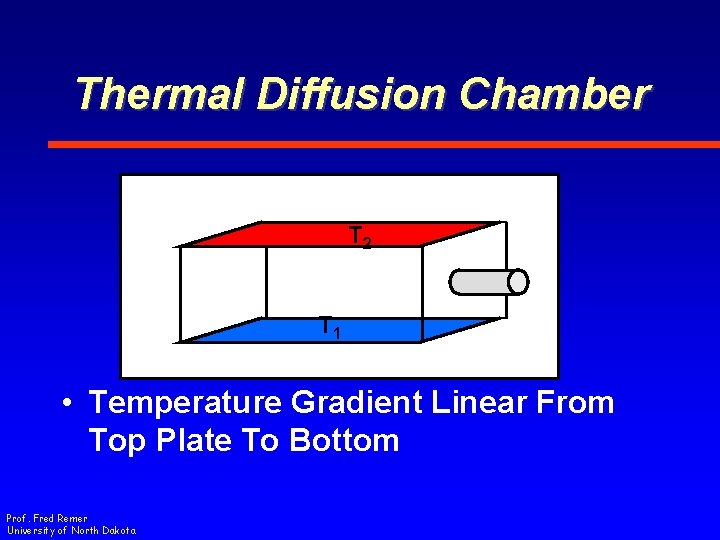
Thermal Diffusion Chamber T 2 T 1 • Temperature Gradient Linear From Top Plate To Bottom Prof. Fred Remer University of North Dakota
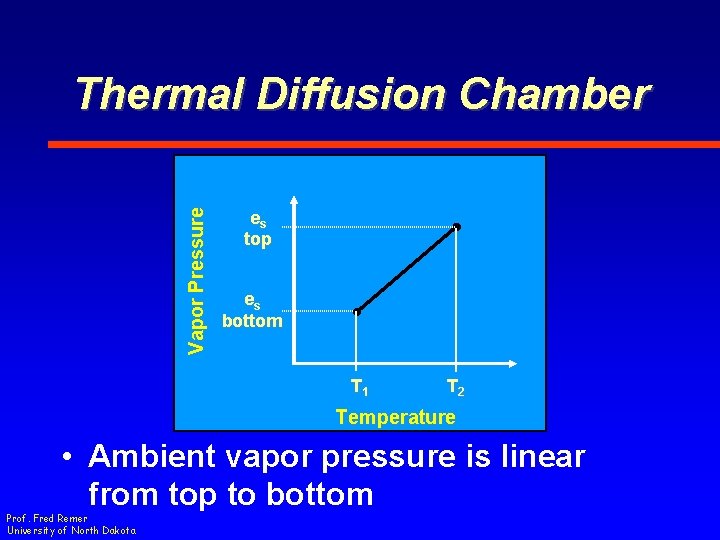
Vapor Pressure Thermal Diffusion Chamber es top es bottom T 1 T 2 Temperature • Ambient vapor pressure is linear from top to bottom Prof. Fred Remer University of North Dakota
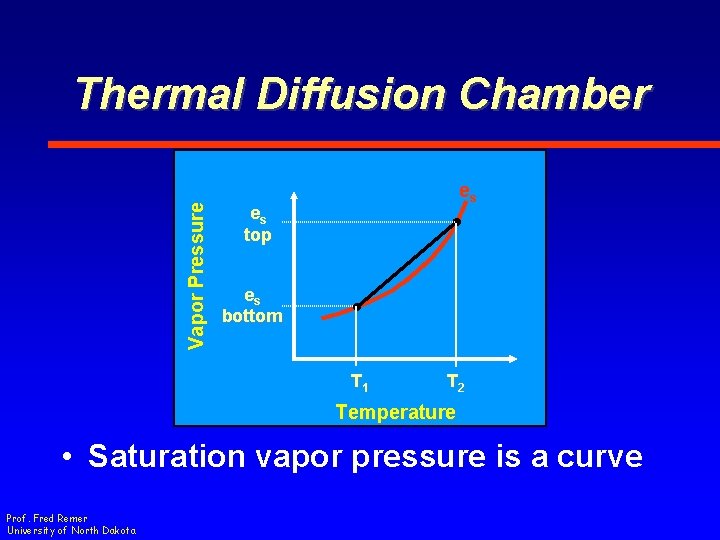
Vapor Pressure Thermal Diffusion Chamber es es top es bottom T 1 T 2 Temperature • Saturation vapor pressure is a curve Prof. Fred Remer University of North Dakota
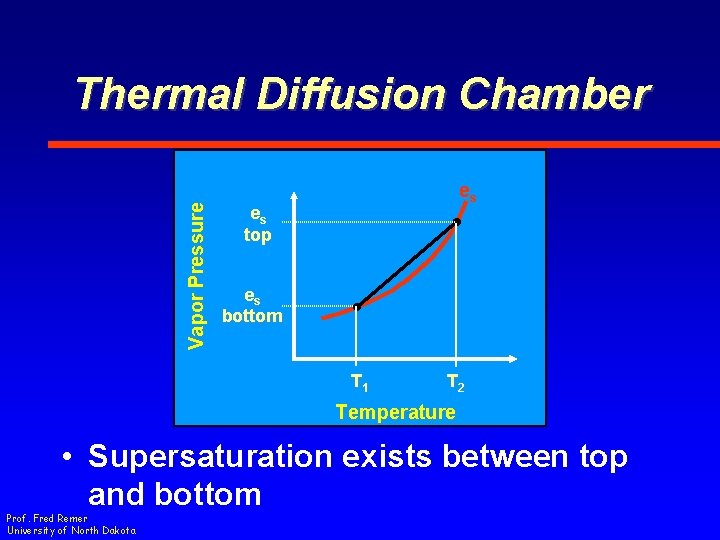
Vapor Pressure Thermal Diffusion Chamber es es top es bottom T 1 T 2 Temperature • Supersaturation exists between top and bottom Prof. Fred Remer University of North Dakota
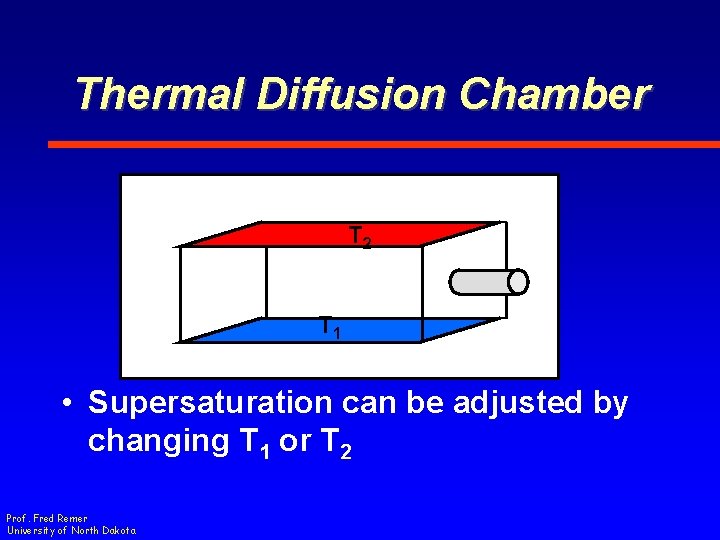
Thermal Diffusion Chamber T 2 T 1 • Supersaturation can be adjusted by changing T 1 or T 2 Prof. Fred Remer University of North Dakota
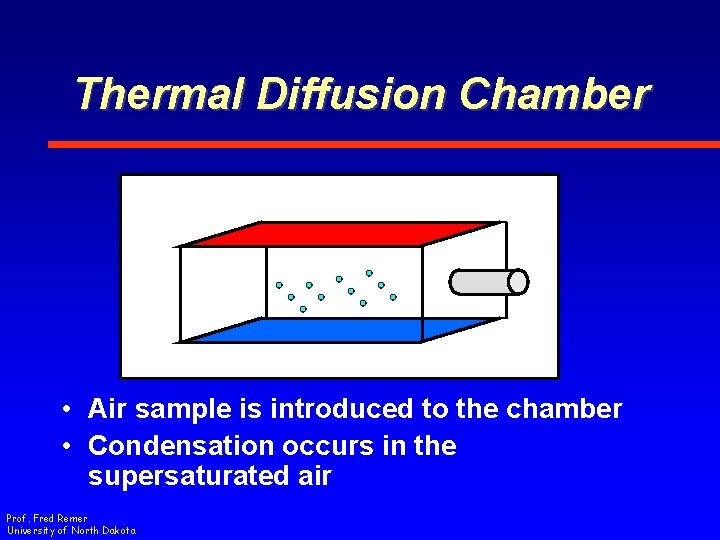
Thermal Diffusion Chamber • Air sample is introduced to the chamber • Condensation occurs in the supersaturated air Prof. Fred Remer University of North Dakota
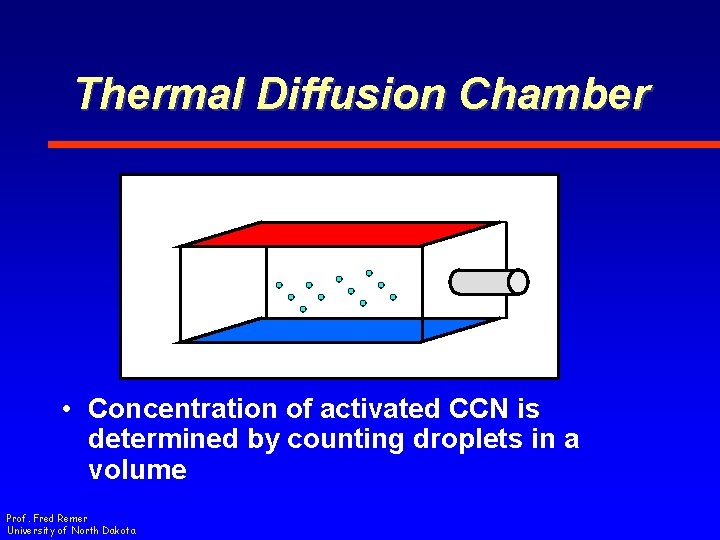
Thermal Diffusion Chamber • Concentration of activated CCN is determined by counting droplets in a volume Prof. Fred Remer University of North Dakota
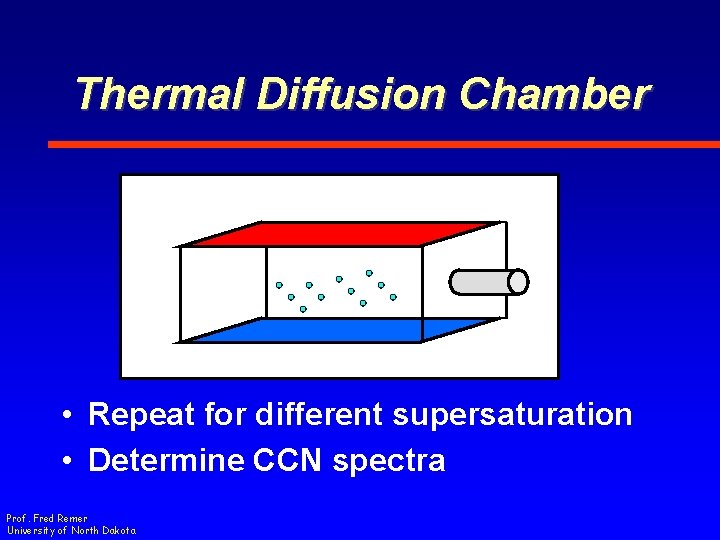
Thermal Diffusion Chamber • Repeat for different supersaturation • Determine CCN spectra Prof. Fred Remer University of North Dakota
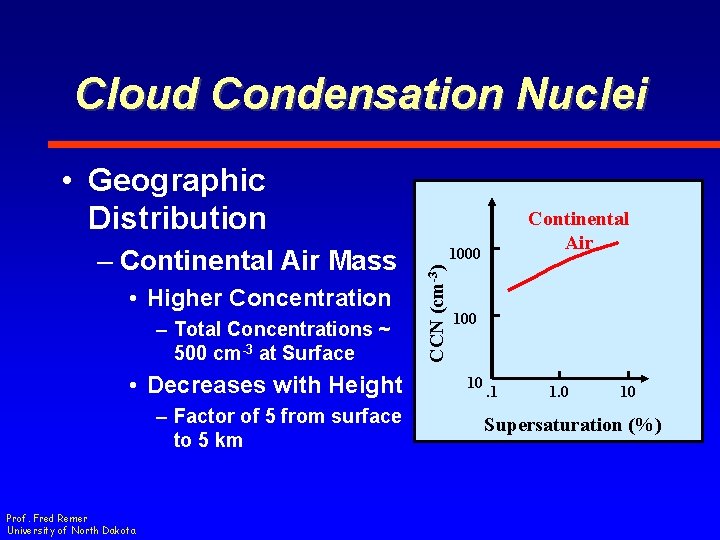
Cloud Condensation Nuclei • Geographic Distribution • Higher Concentration – Total Concentrations ~ 500 cm-3 at Surface • Decreases with Height – Factor of 5 from surface to 5 km Prof. Fred Remer University of North Dakota 1000 CCN (cm-3) – Continental Air Mass Continental Air 100 10 . 1 1. 0 10 Supersaturation (%)
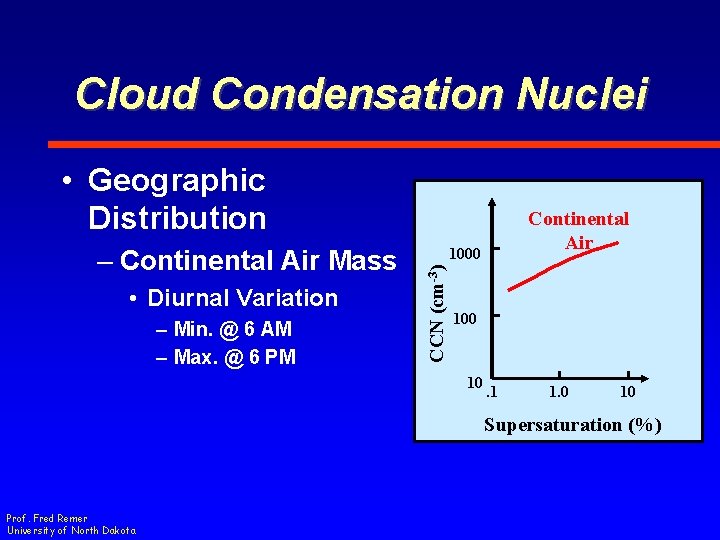
Cloud Condensation Nuclei • Geographic Distribution • Diurnal Variation – Min. @ 6 AM – Max. @ 6 PM 1000 CCN (cm-3) – Continental Air Mass Continental Air 100 10 . 1 1. 0 10 Supersaturation (%) Prof. Fred Remer University of North Dakota
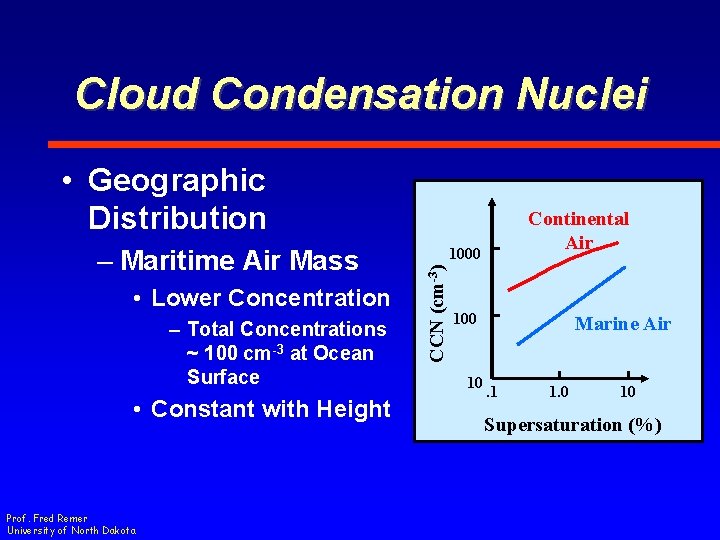
Cloud Condensation Nuclei • Geographic Distribution • Lower Concentration – Total Concentrations ~ 100 cm-3 at Ocean Surface • Constant with Height Prof. Fred Remer University of North Dakota 1000 CCN (cm-3) – Maritime Air Mass Continental Air 100 10 Marine Air. 1 1. 0 10 Supersaturation (%)
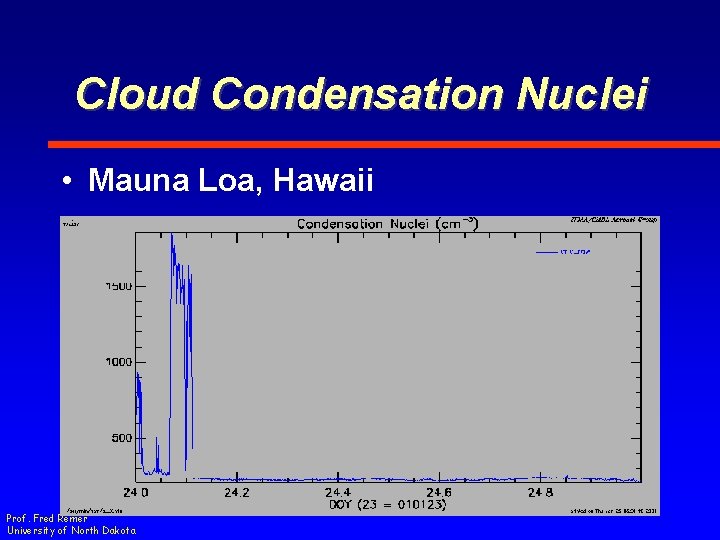
Cloud Condensation Nuclei • Mauna Loa, Hawaii Prof. Fred Remer University of North Dakota
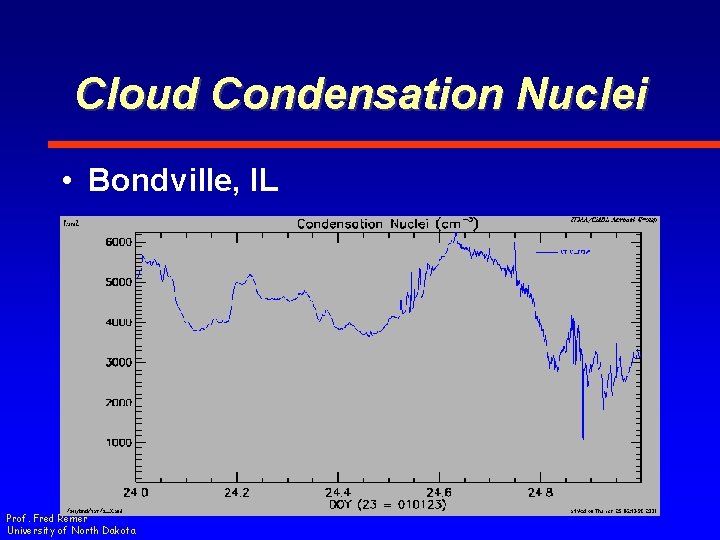
Cloud Condensation Nuclei • Bondville, IL Prof. Fred Remer University of North Dakota
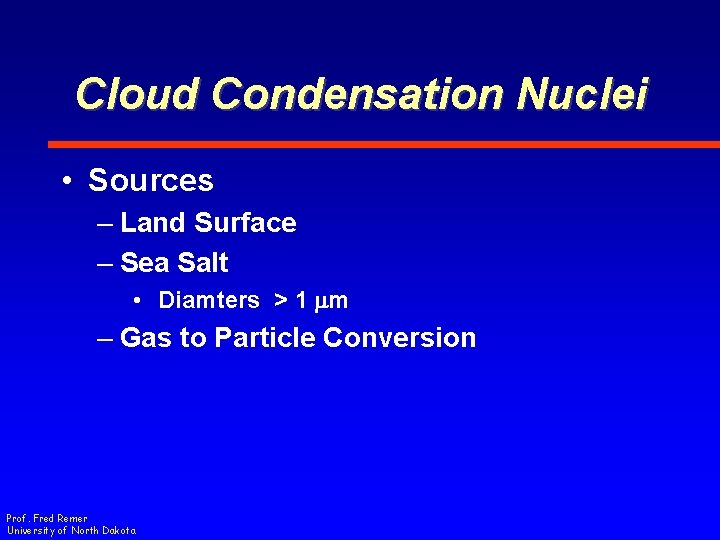
Cloud Condensation Nuclei • Sources – Land Surface – Sea Salt • Diamters > 1 mm – Gas to Particle Conversion Prof. Fred Remer University of North Dakota
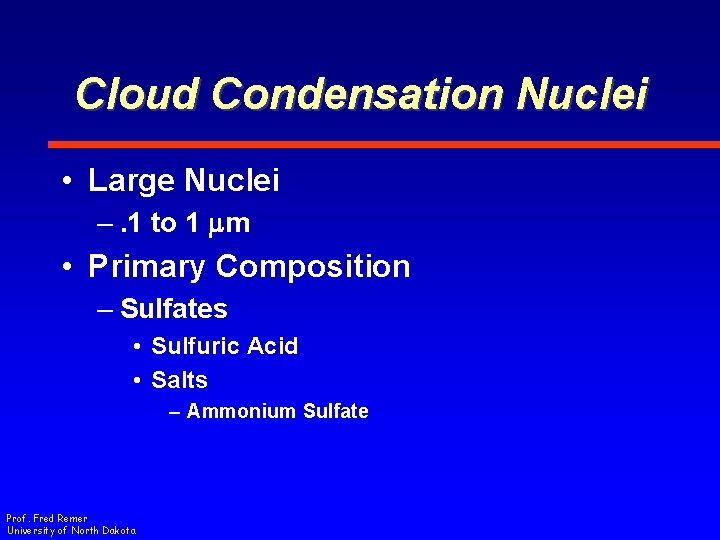
Cloud Condensation Nuclei • Large Nuclei –. 1 to 1 mm • Primary Composition – Sulfates • Sulfuric Acid • Salts – Ammonium Sulfate Prof. Fred Remer University of North Dakota
What are cloud droplets
Earth science reference table dew point
Calculate relative humidity
Relative humidity chart
Weather station model symbols
Relative humidity
Relative humidity on a station model
Krystal crystallizer working
Miers supersaturation theory
Krystal crystallizer
Supersaturation definition
Which of the three blood droplets shown
Droplets
Droplets
Ladder graphic organizer
Evaporation
Check liste formation prof
Formation initiale vs formation continue
What is humidity
Humidity recorder turkey
Melia sehat sejahtera
Identify three effects of low humidity s190
High humidity meaning
Outlook temperature humidity wind play
What is humidity
What is humidity
What happens when the temperature of the air cools brainpop
Three redwood trees are kept at different humidity levels
Humidity 2 optimization
Saturation and condensation
Percolation vs infiltration
Absolute humidity
Humidity and meniere's disease
Contoh soal psychrometric chart
Absolute humidity
Humidity
Which statement accurately describes humidity?
Which phrase best describes coal?
Humidity server room
Humidity evaporation
Bland aerosol therapy
Relative clauses and relative pronouns stage 15
What is a conditional relative frequency
The person who phoned me last night is my teacher
Relative adverb sentences
Steps for cloud formation
Morning glory cloud formation
Cloud formation steps
Formation cloud
Section 18.2 cloud formation
Curvature effect in cloud formation
Cloud type
What does nimbo mean when added to a cloud name
Lesson 2 clouds
Cloud formation
Pixabay
A composable component must be modular
Cloud integration patterns
Public cloud vs private cloud cost analysis
Snapcloud
Prof. dr. marcus eckert
Agamenon roberto
Prof david toback
Prof dr ali hossain
Prof suganda tanuwidjaja
Th
Le slide della prof di lettere e sostegno
Conclusion partielle texte argumentatif exemple
Rodzaj i gatunek syzyfowe prace
Cua study abroad
Sonnet 29 by edna analysis
Akuisita
Nadp redüktaz
Obsatar sinaga
Keylosis
Prof mark ferguson
Prof paolo bellioni
Ramlimusa.com
Prof aris sudiyanto
Prof agamenon roberto
Bilgin arda
Epinoryum
Prof jusak nugraha
Umi narimawati
Prof dr hayri ülgen
Prof tan malaka
Parenteral beslenme kontrendikasyonları
Prof. dr. fevziye toros
Ledo vacarro
Prof tomasz targowski
Prof dr yahya büyükaşık
Prof msc
Dr jelena drulovic
Prof. dr. jan papy
Prof. dr mehmet yilmazer
Mikrochimeryzm płodowo matczyny
Sweep line algorithm cp algorithm
Prof fabrizio onida
Comment remercier pour un stage
Prof. norbert pohlmann
Hunner ülseri
Prof. mirosław krajewski
Dr svetlana stanišić
Solcellepanel oppbygging
Fsh valori
Prof dr mualla polat
Vedopo
Prof. dr biljana stojković
özgür akbayır
Prof dr mustafa şahin endokrin
Ayah dr. iris rengganis
Dr martin rumende
Kenantis
Inserra gaetano gastroenterologo catania
Under supervision of prof
Prof cybelle
Pity me not sonnet 29
Adenin
Prof. dr. qumrul ahsan
Prof qomariyatus sholihah
Bbrek
Wiwi prüfungstermine upb