4 Cells The Working Units of Life Chapter
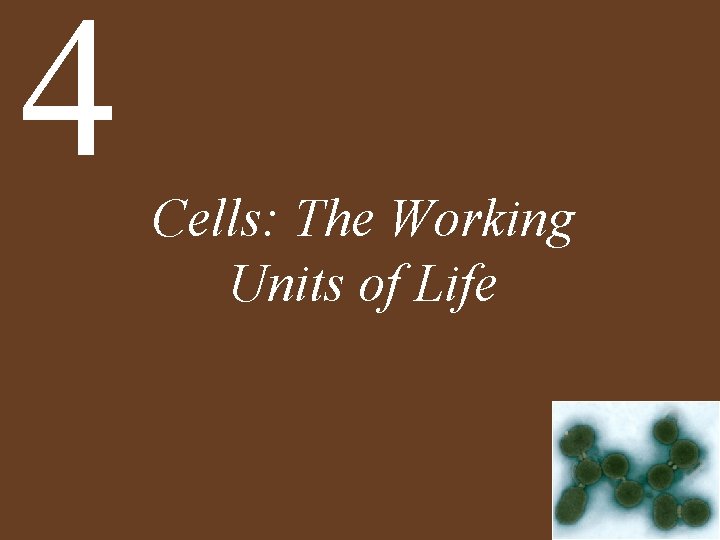
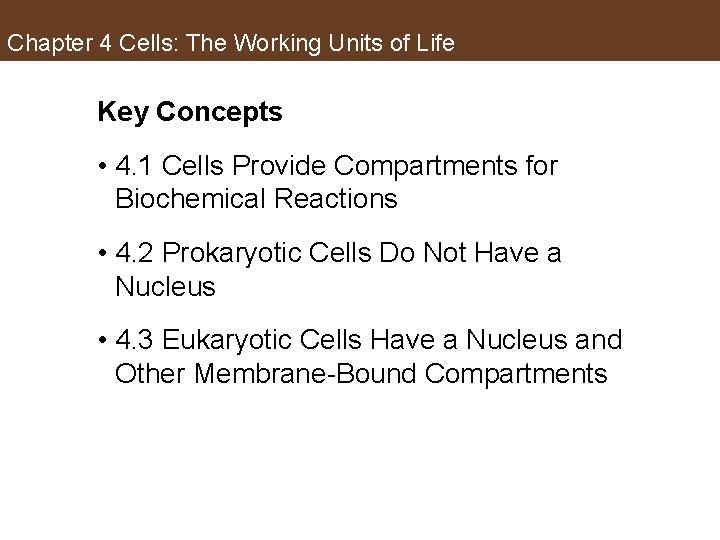
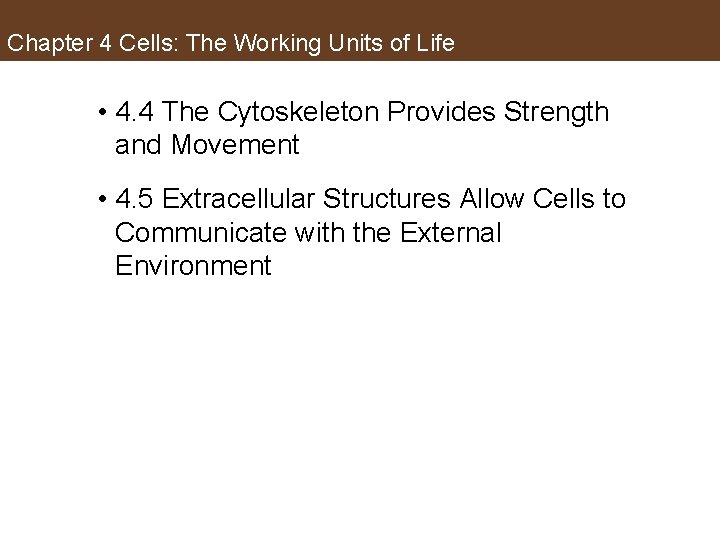
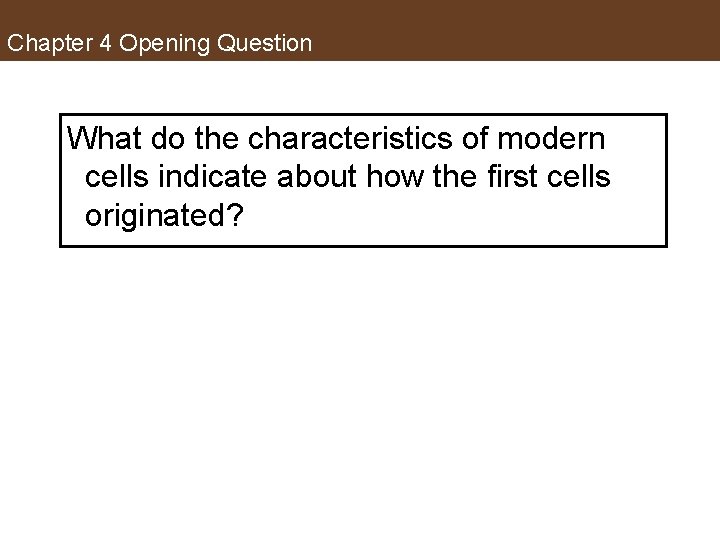
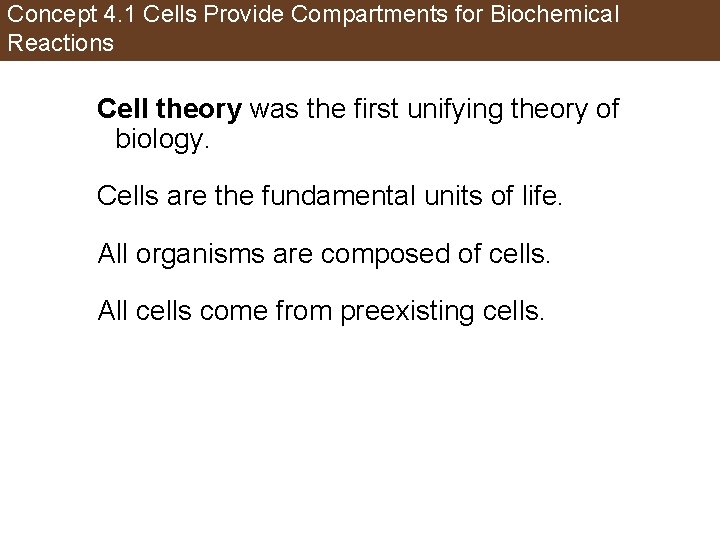
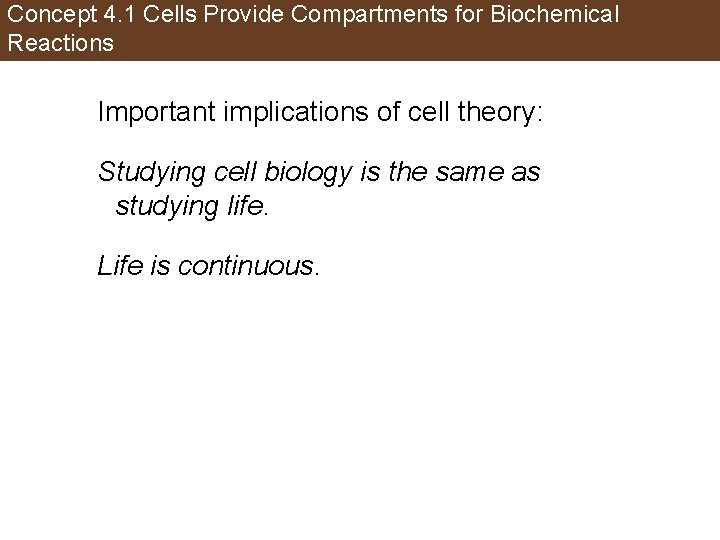
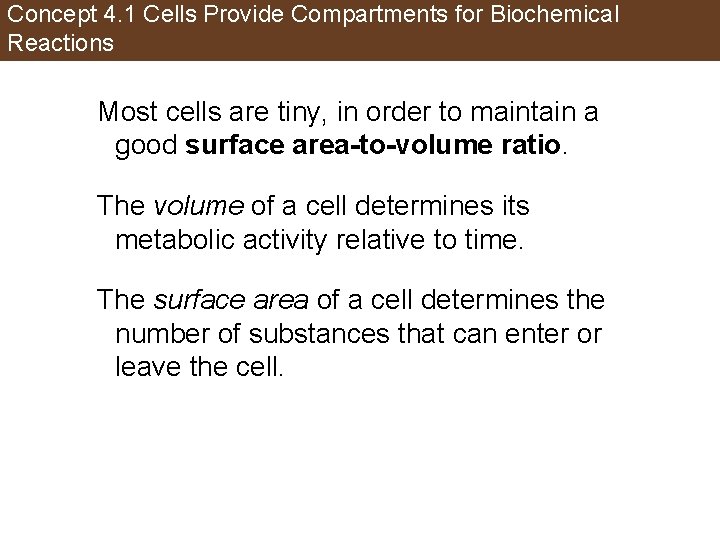
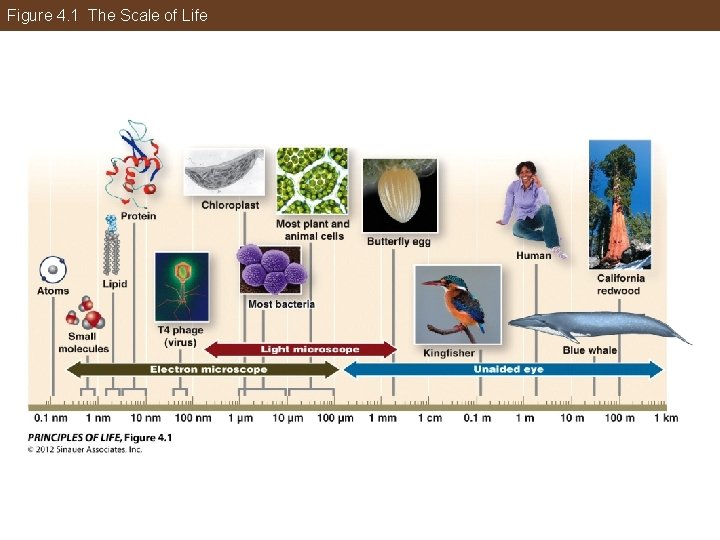
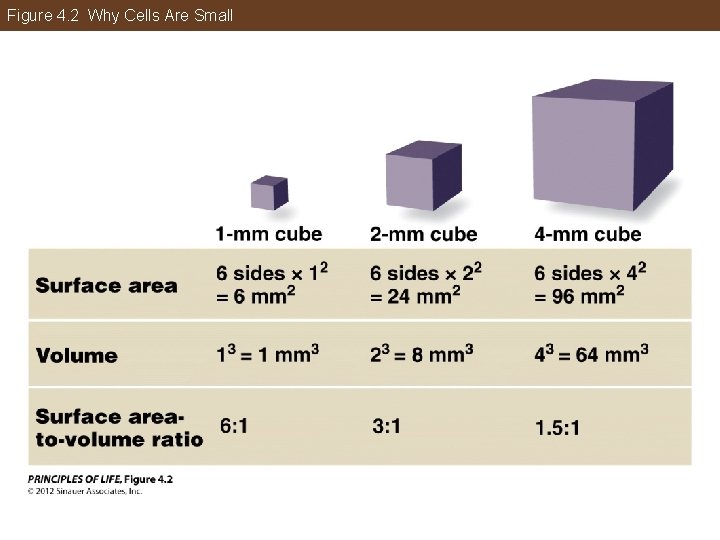
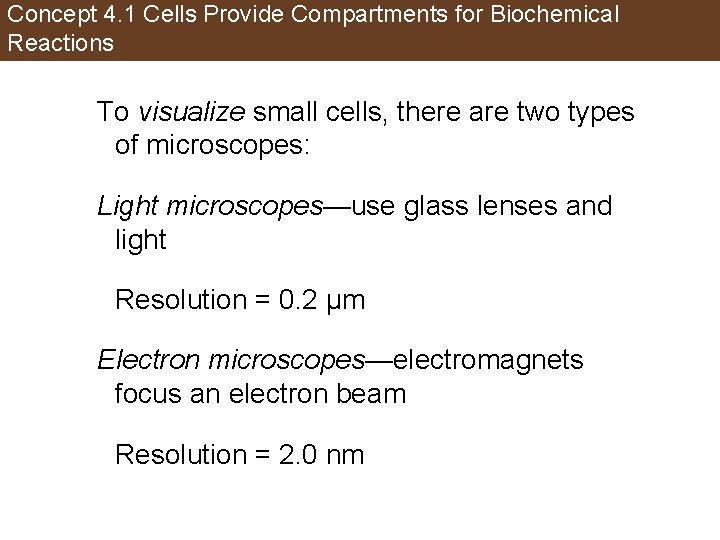
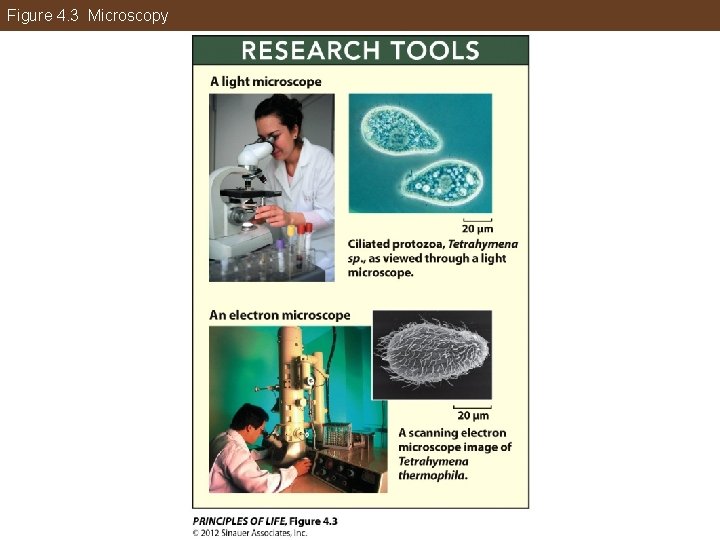
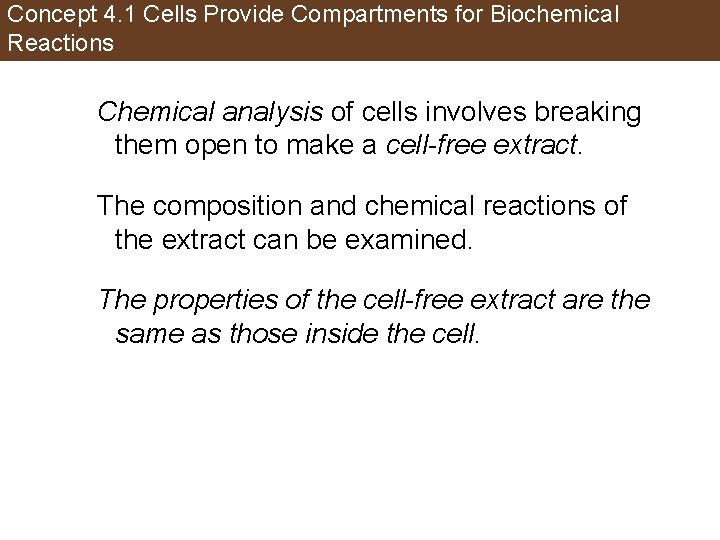
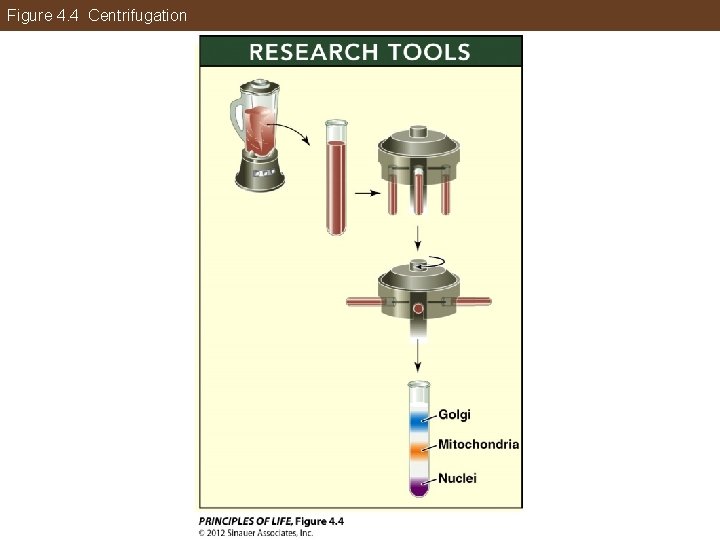
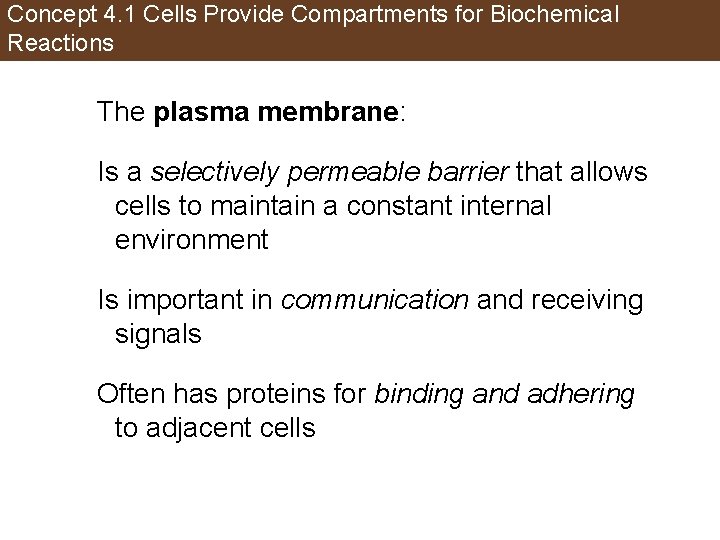
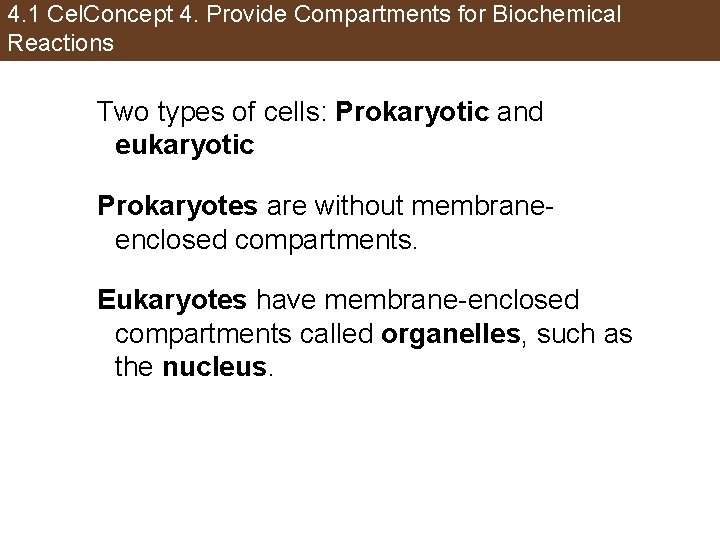
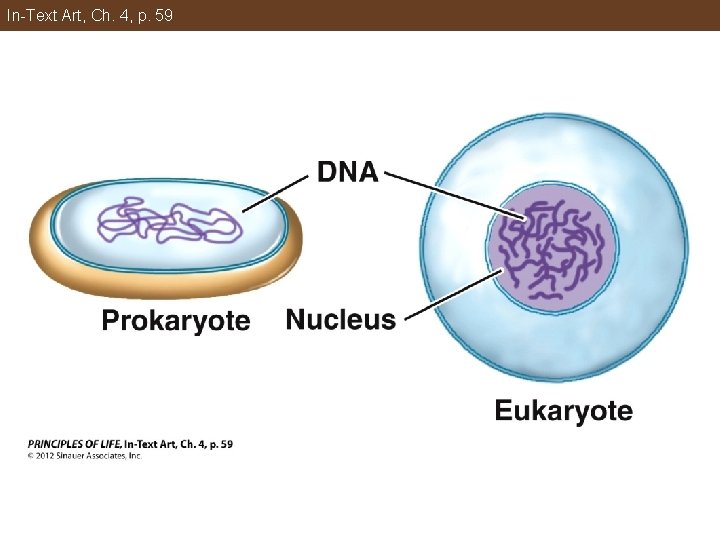
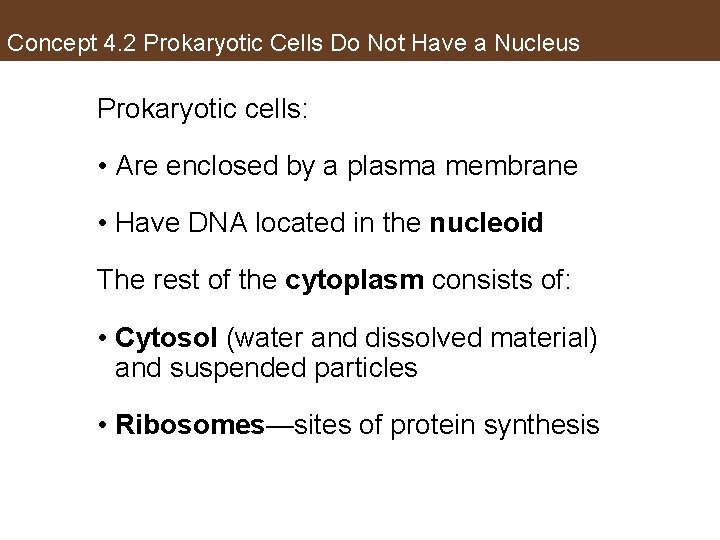
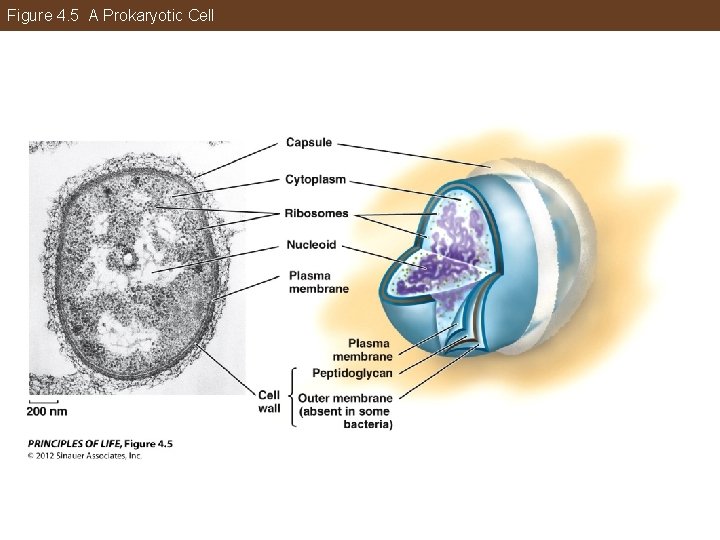
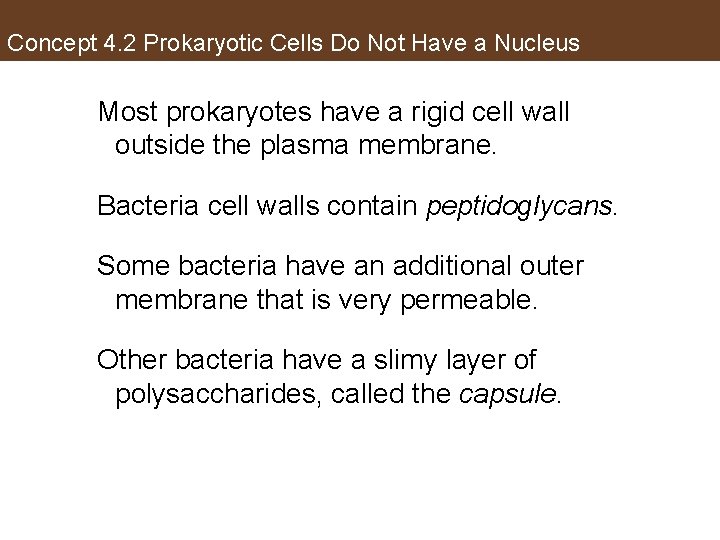
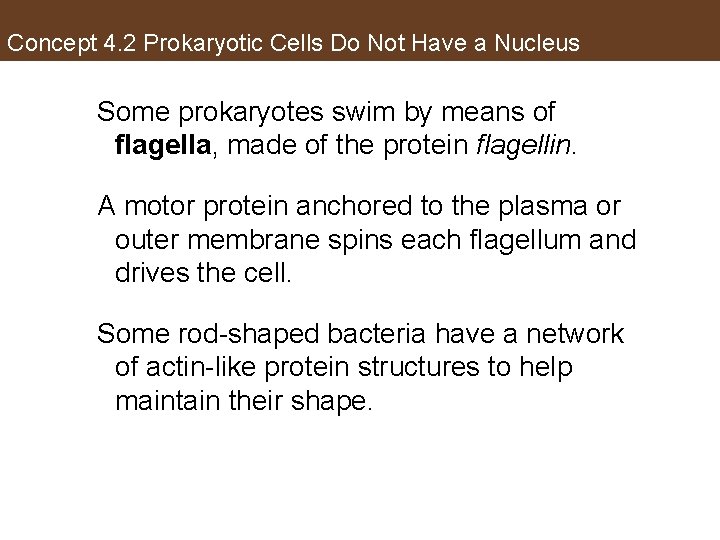
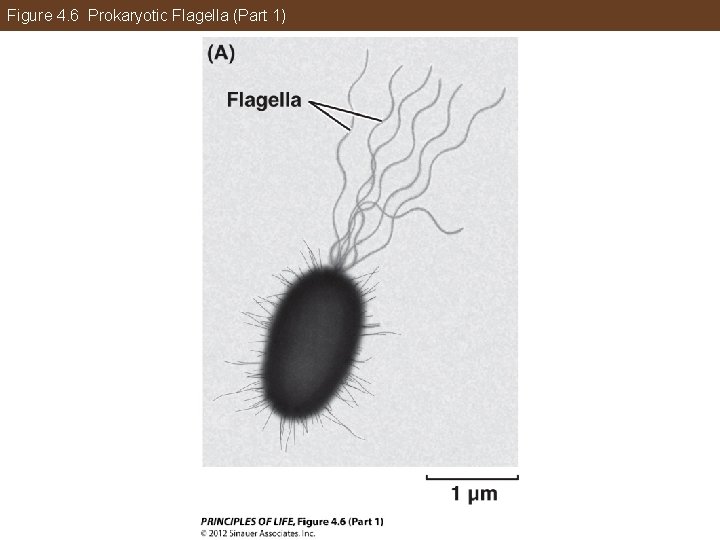
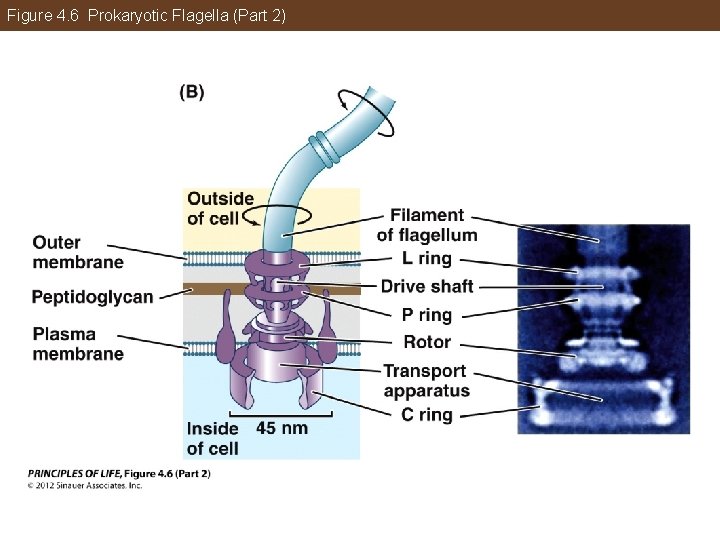
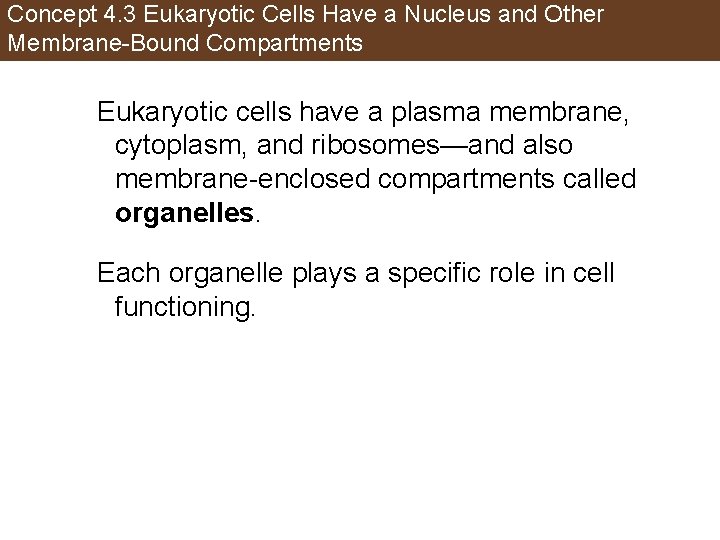
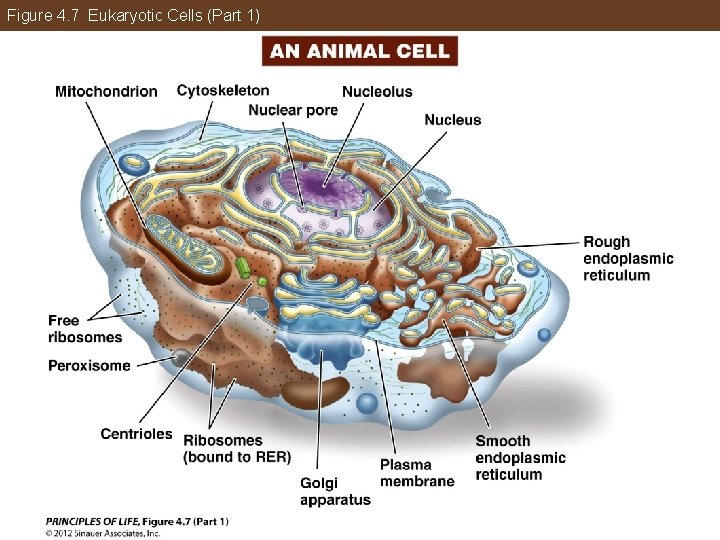
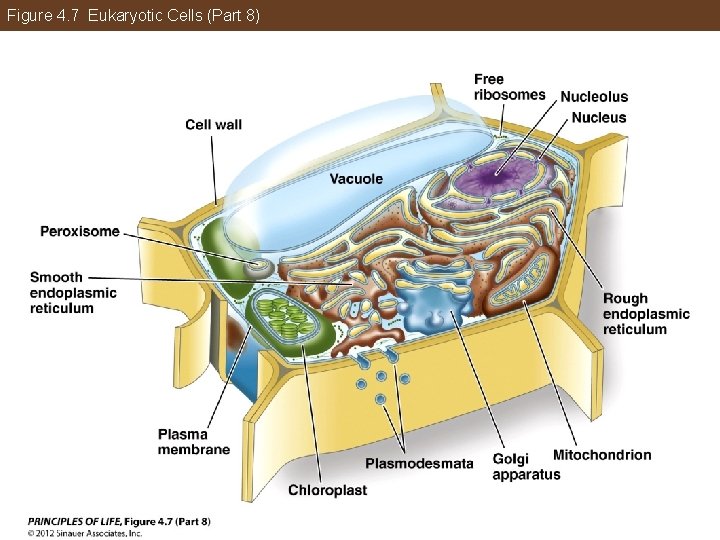
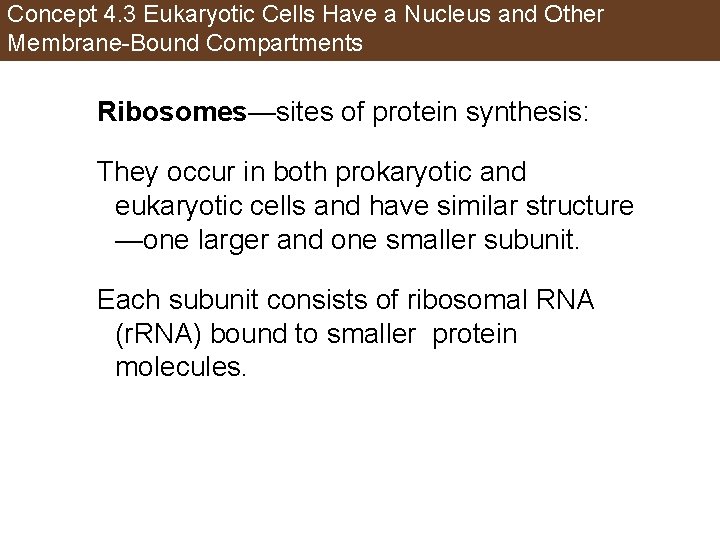
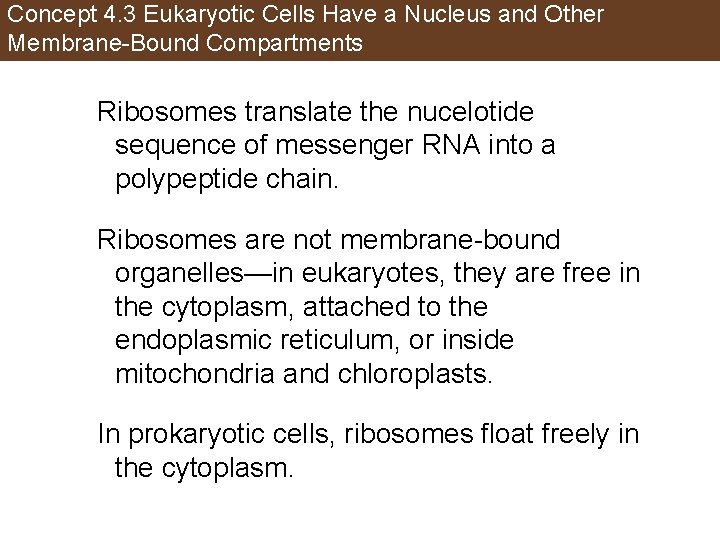
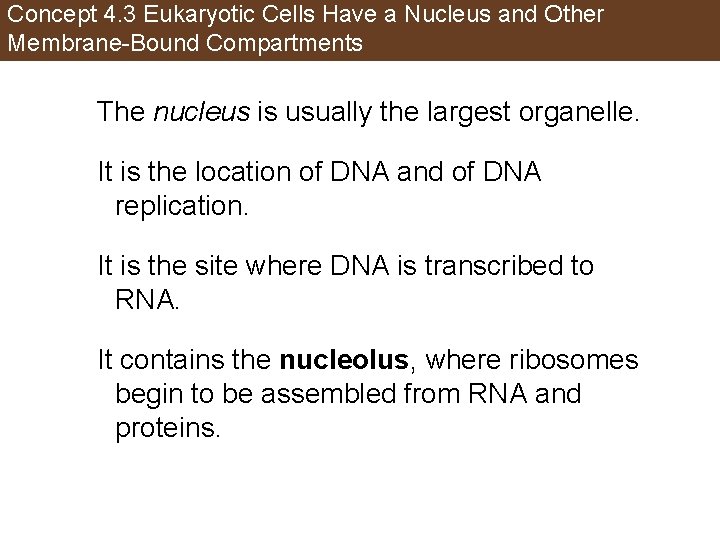
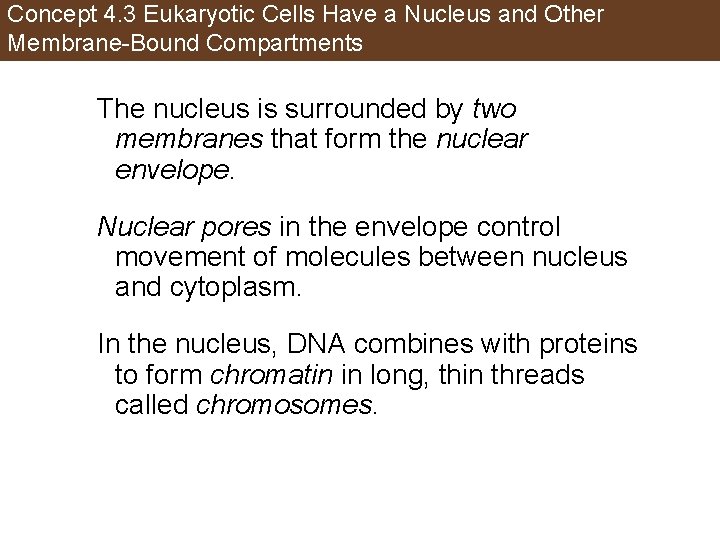
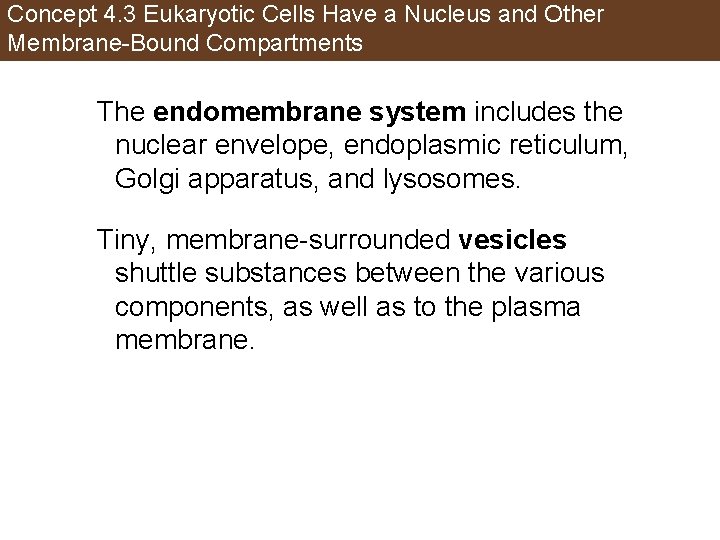
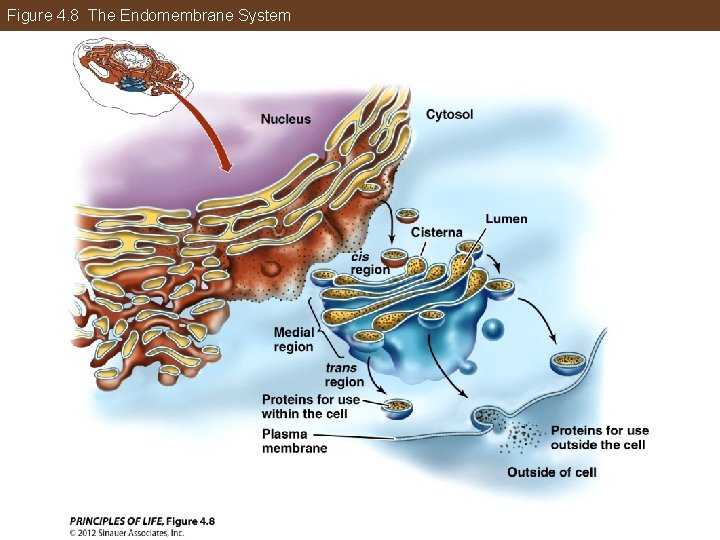
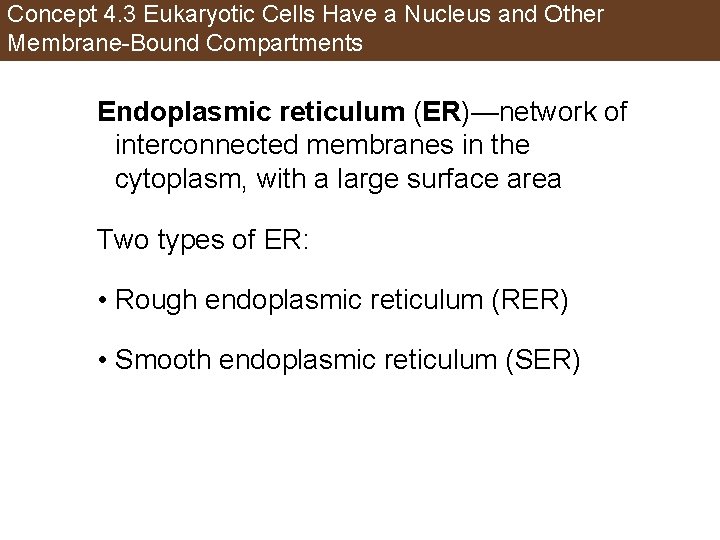
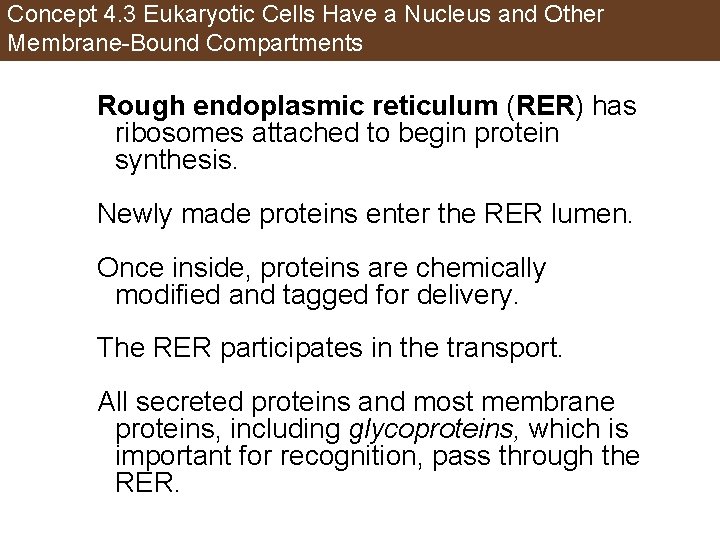
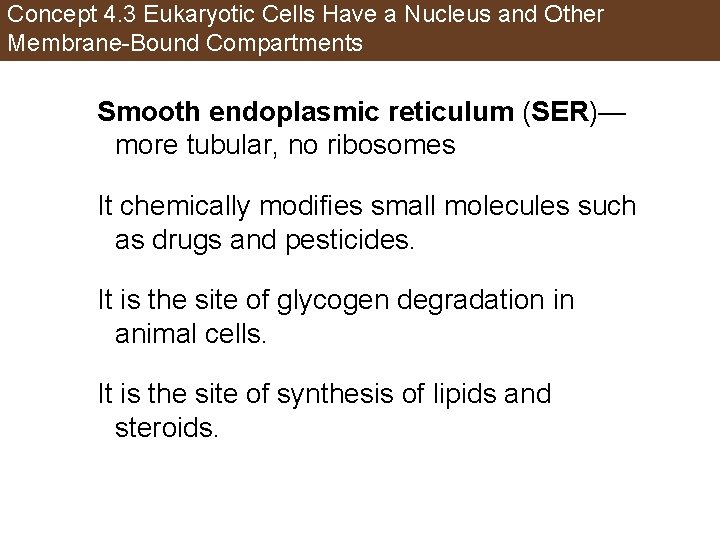
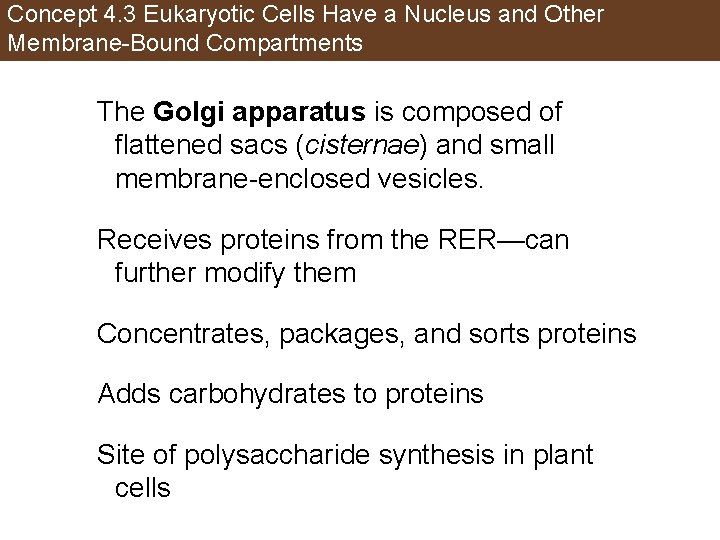
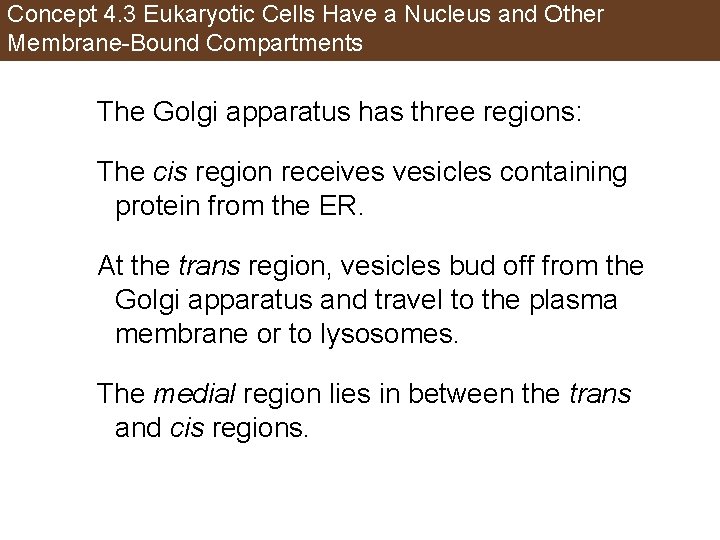
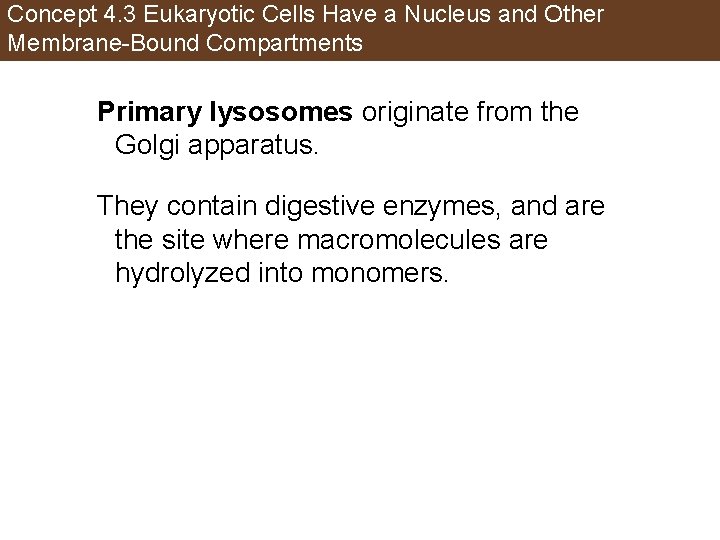
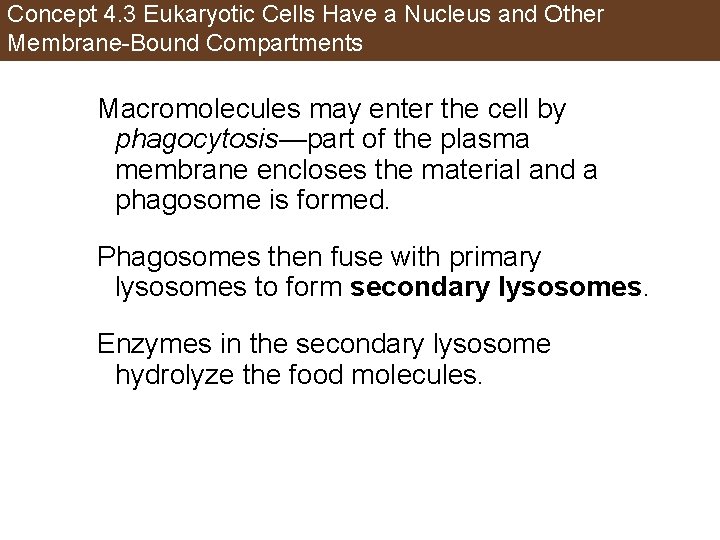
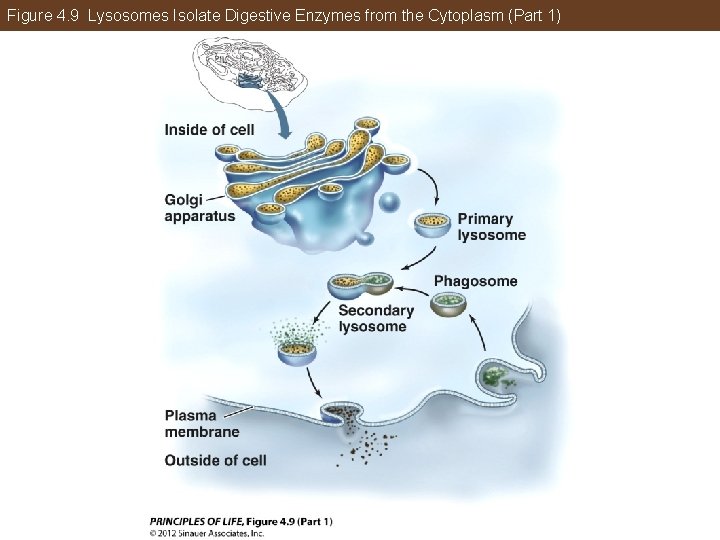
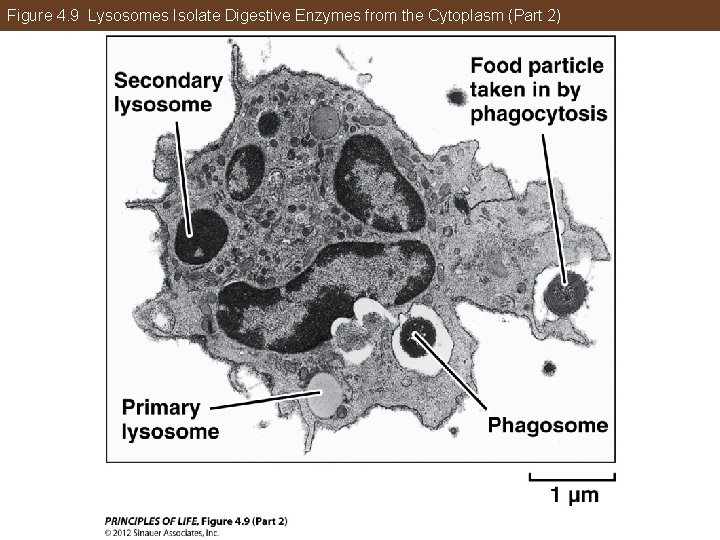
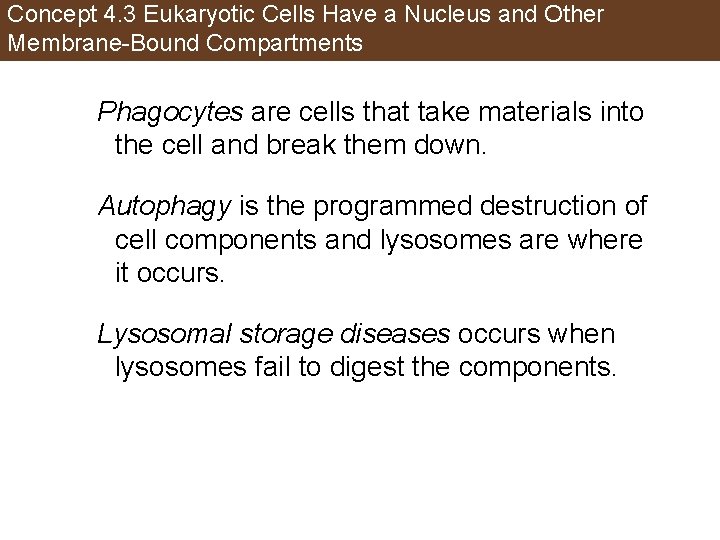
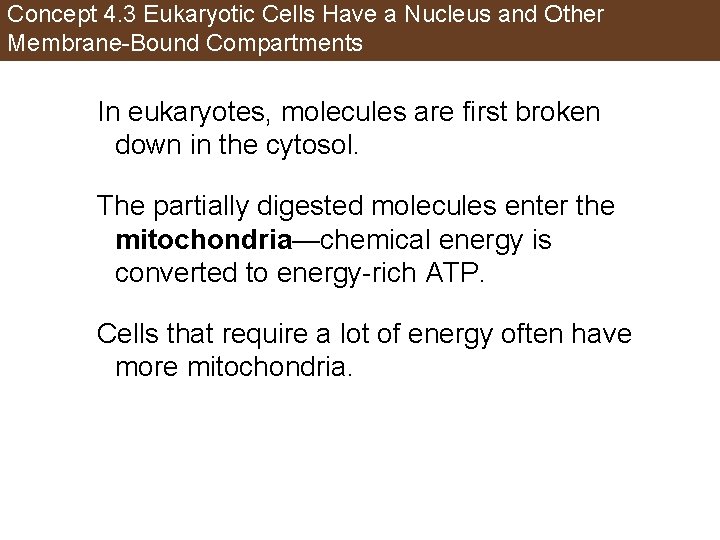
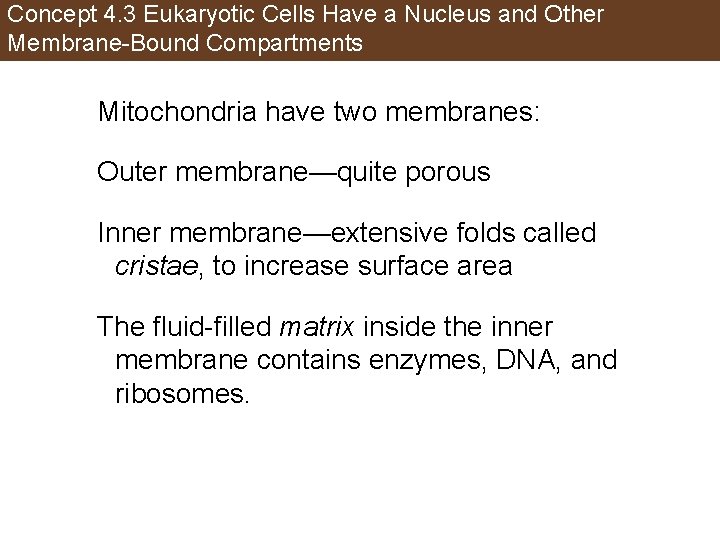
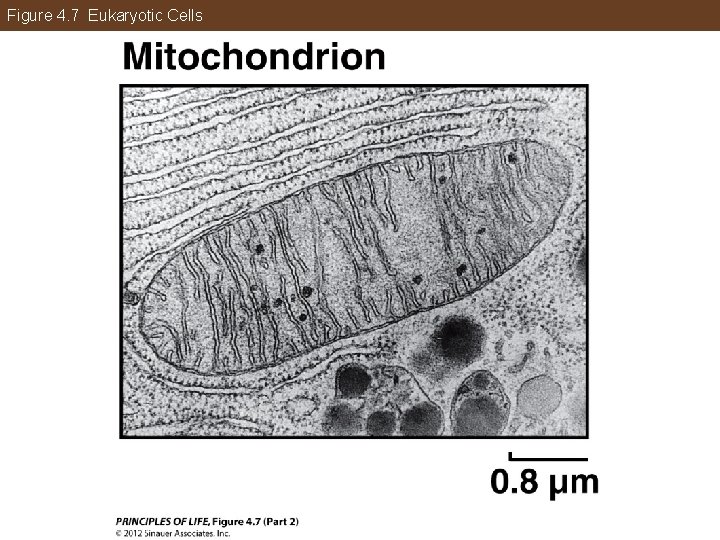
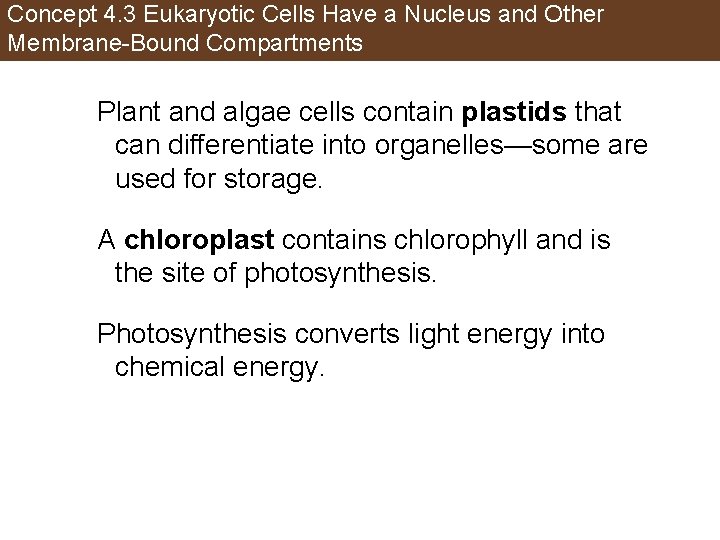
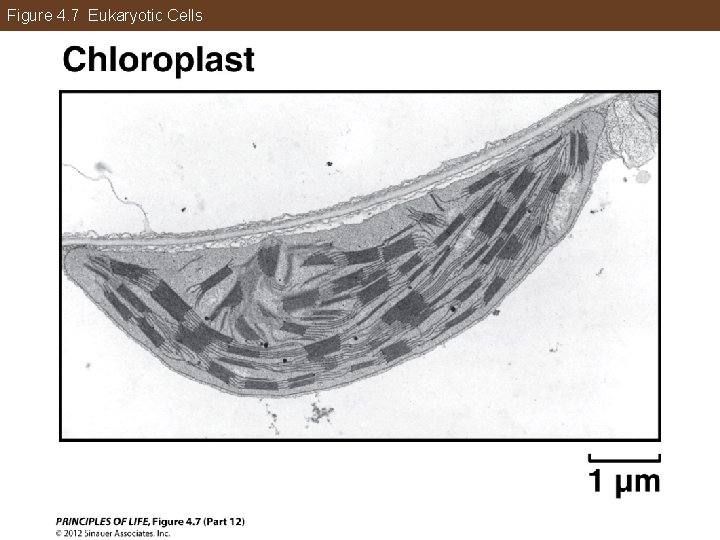
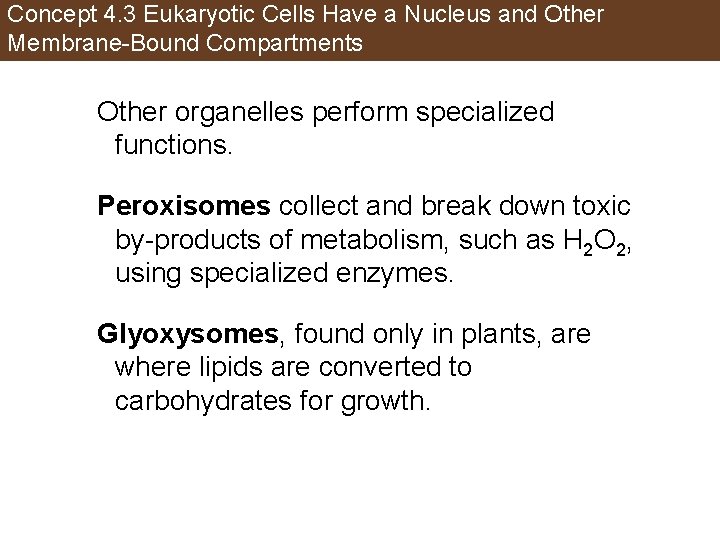
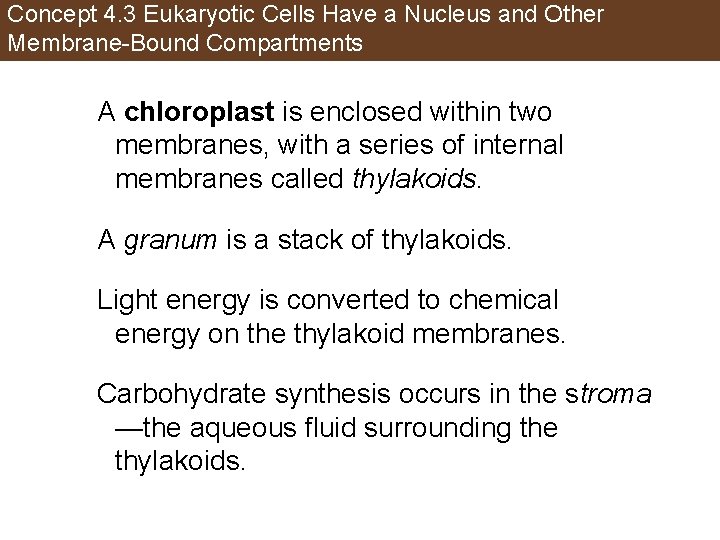
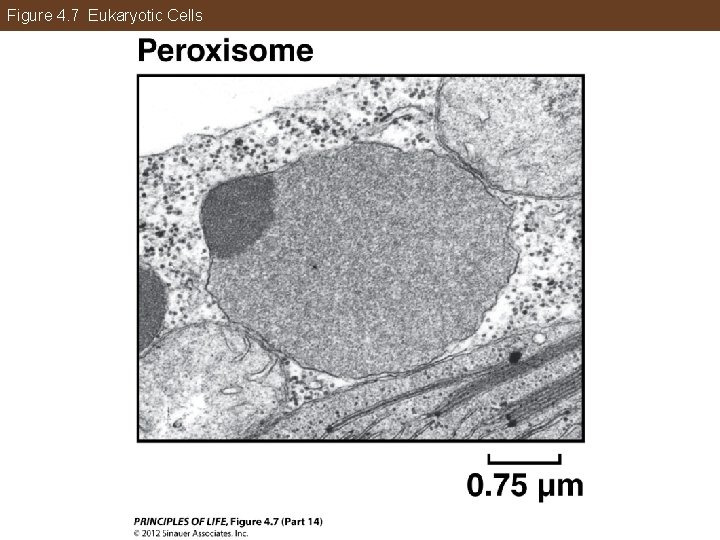
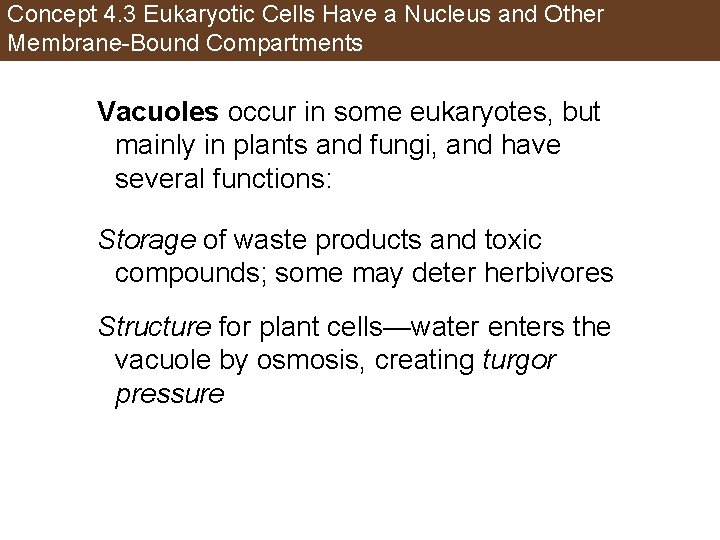
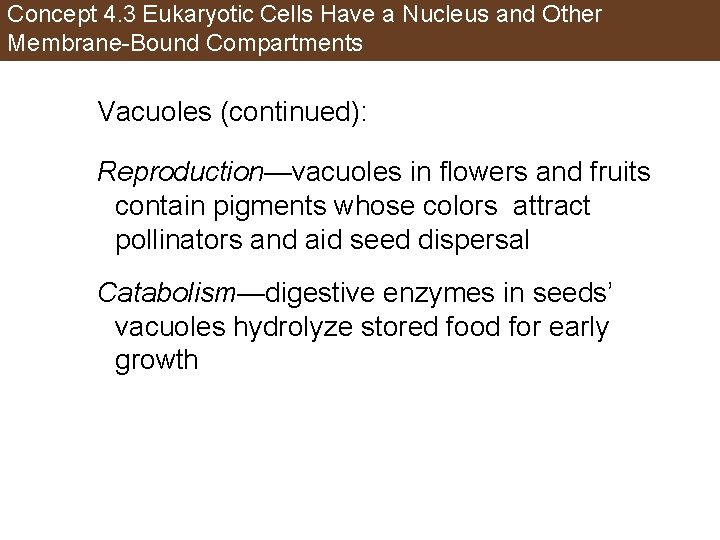
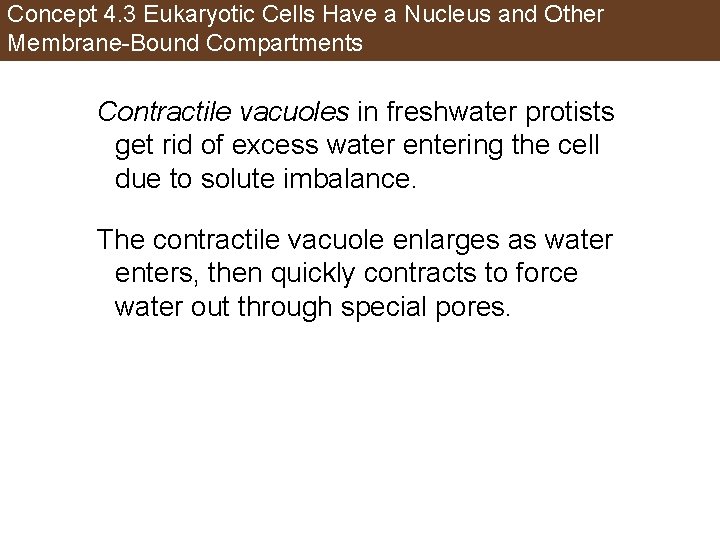
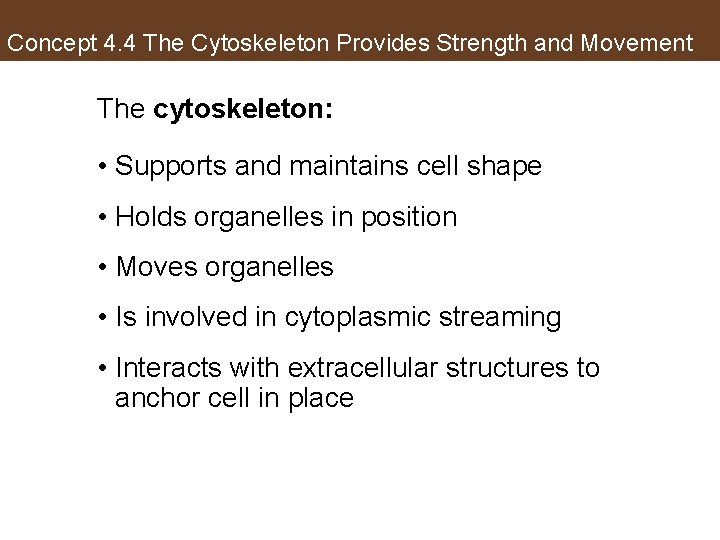
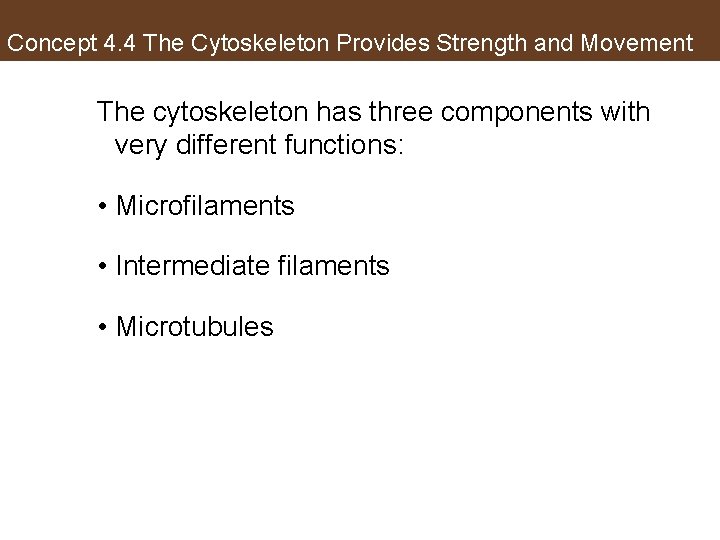
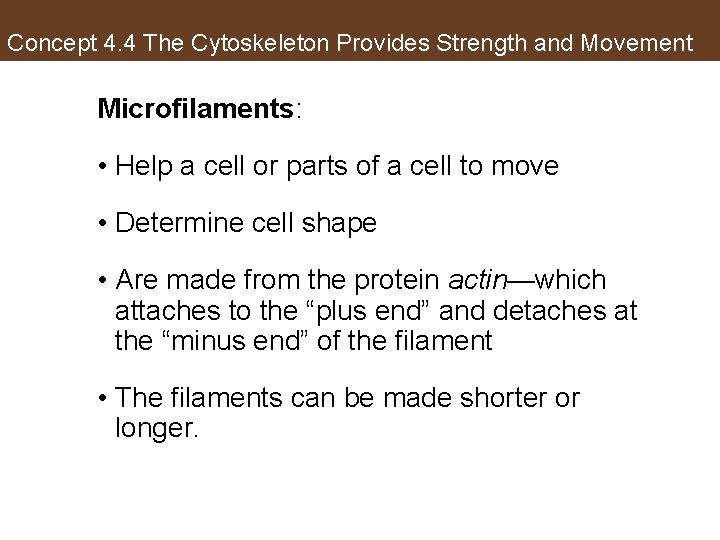
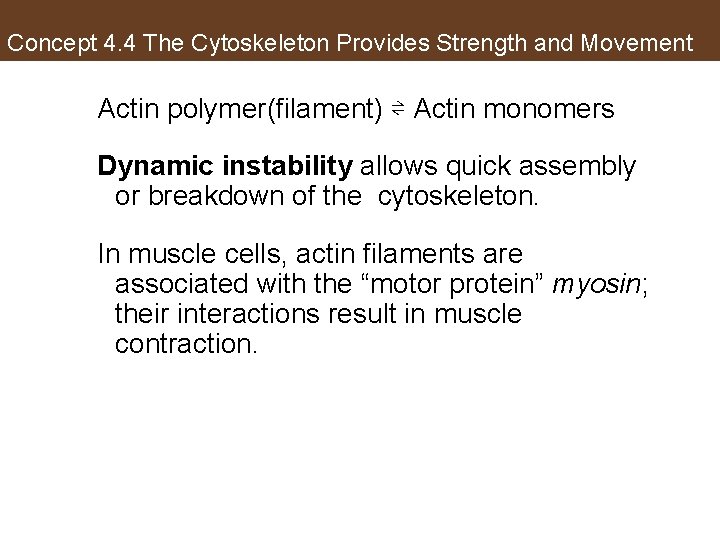
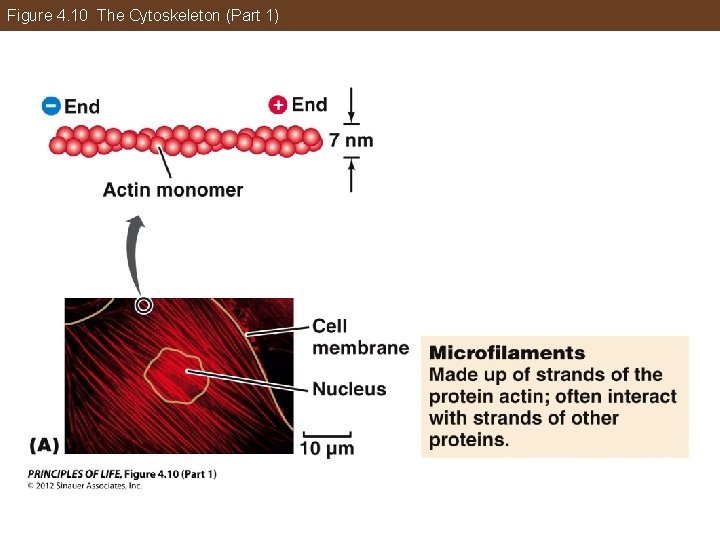
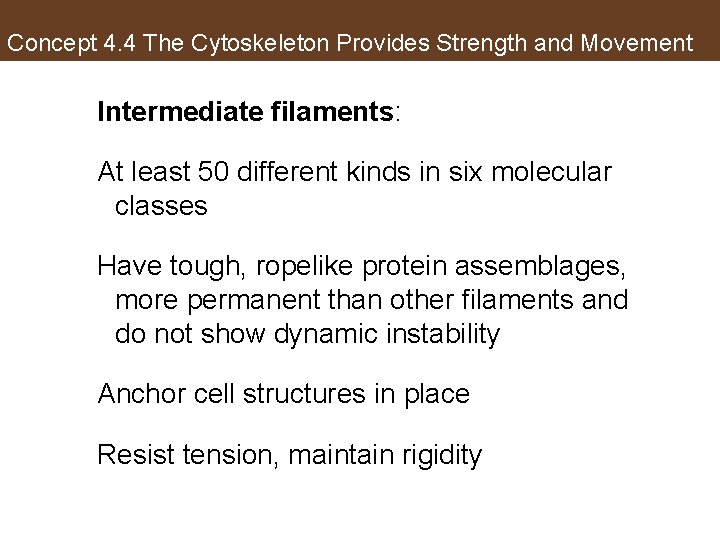
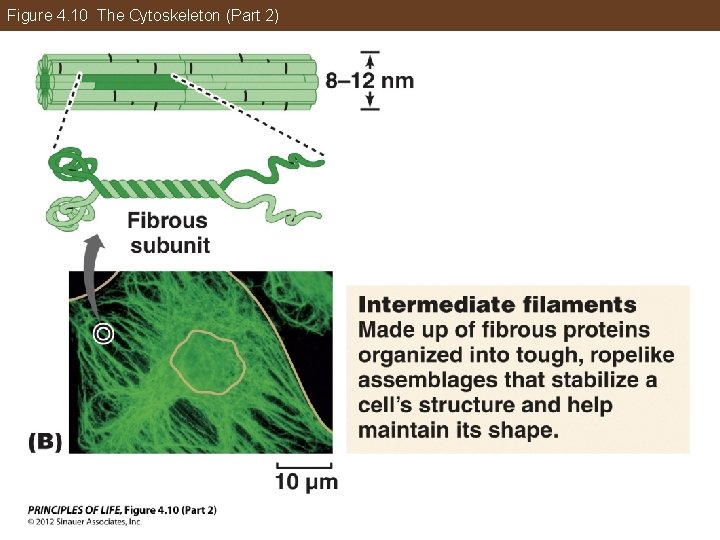
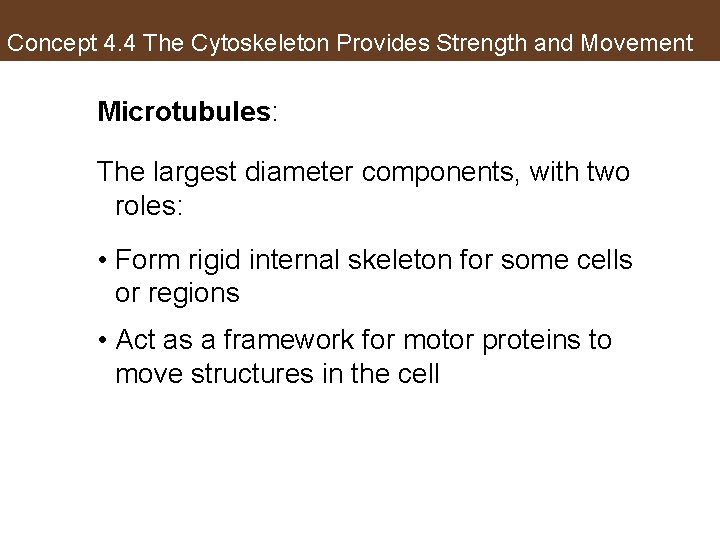
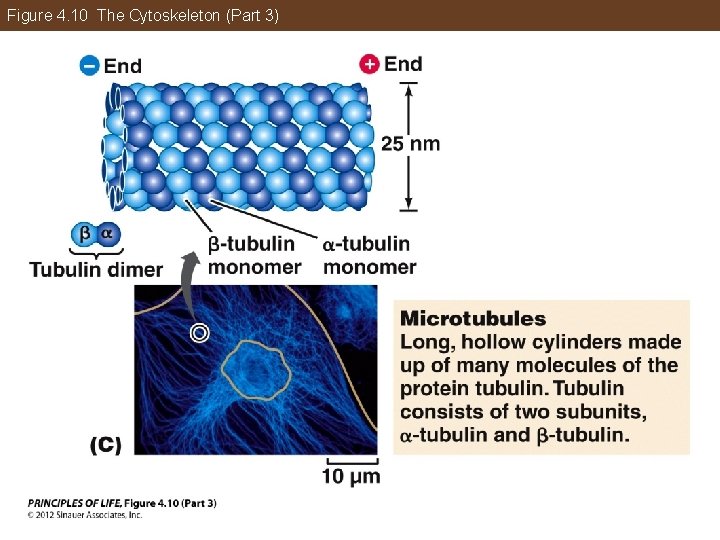
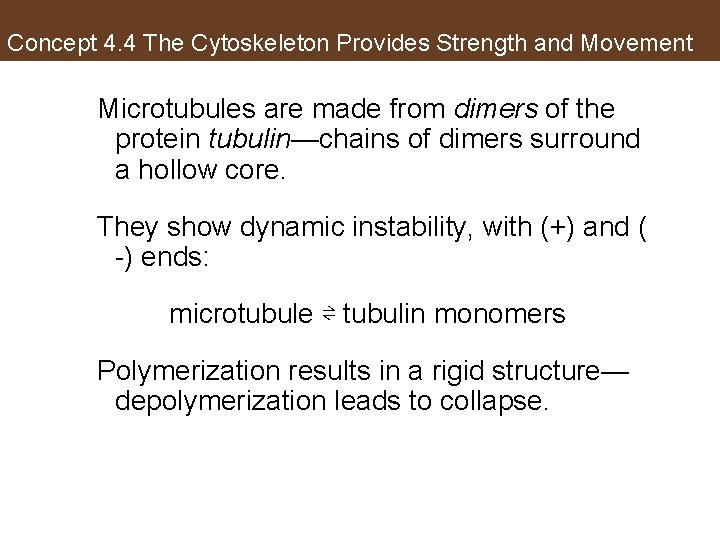
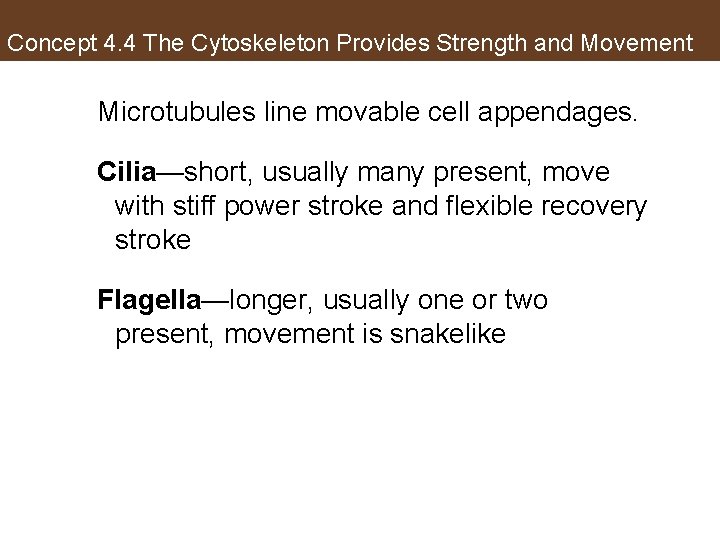
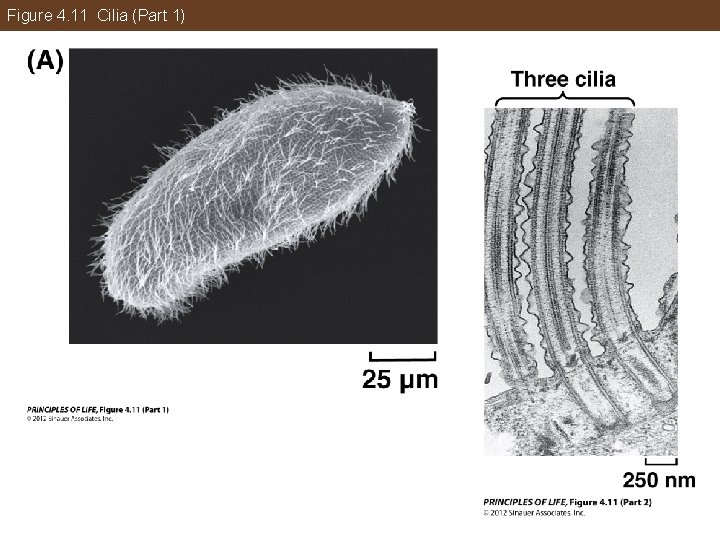
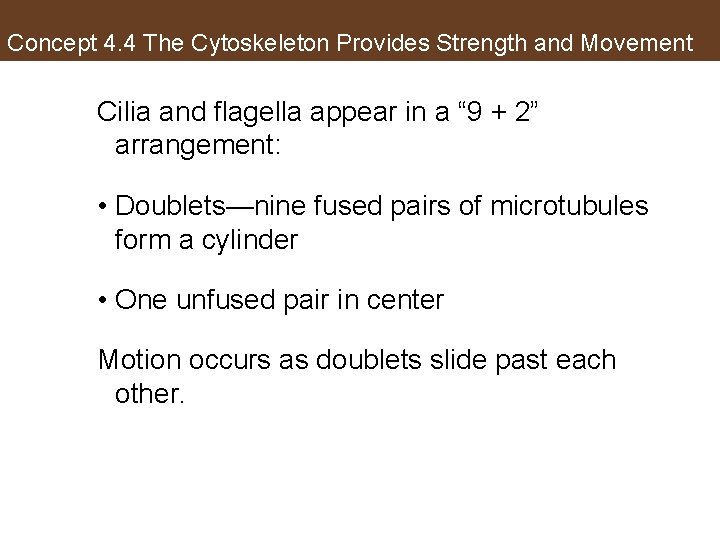
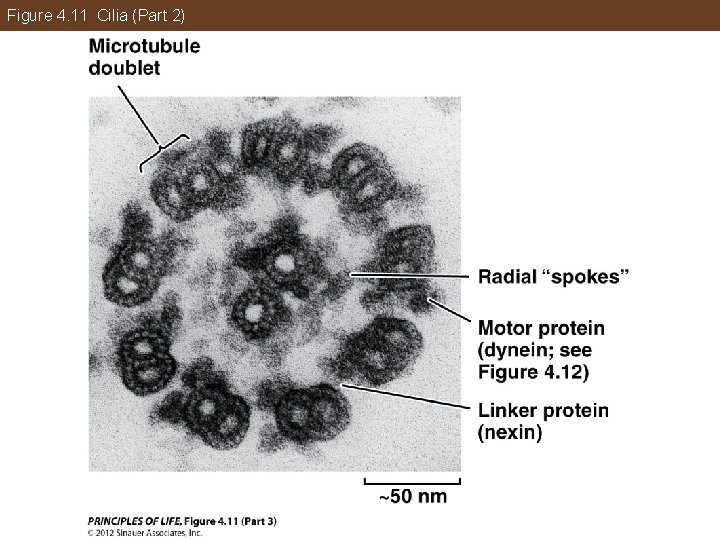
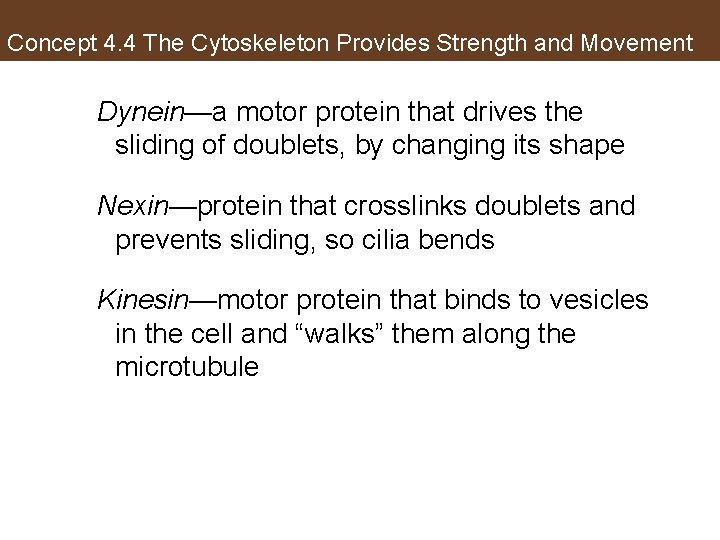
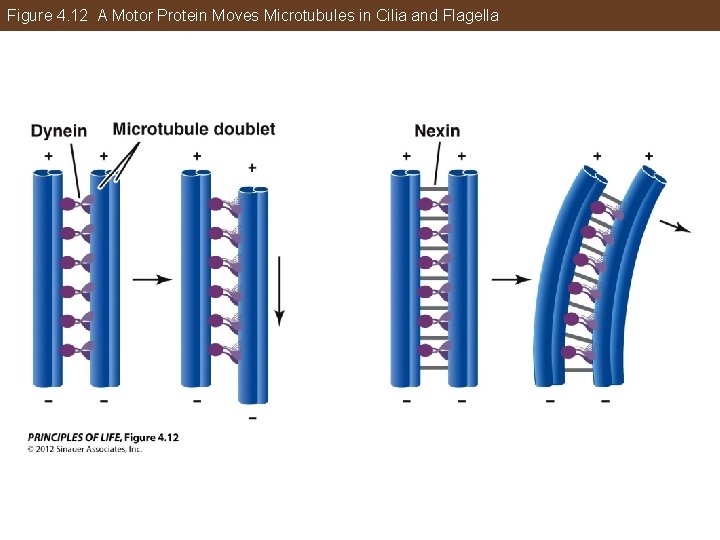
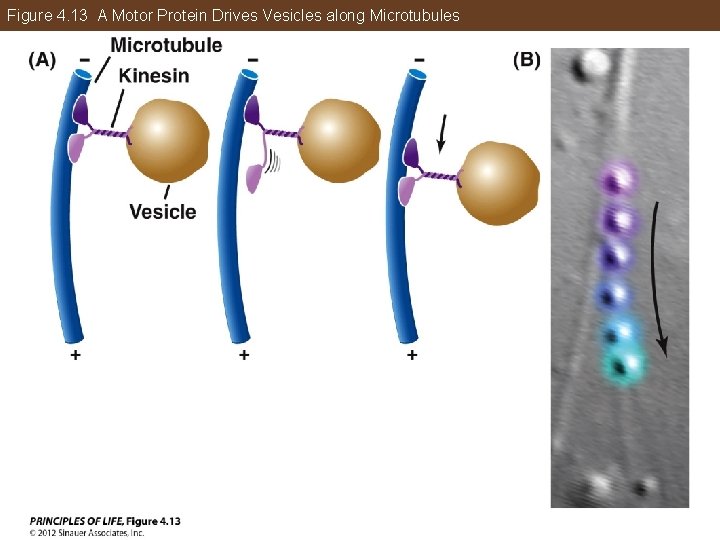
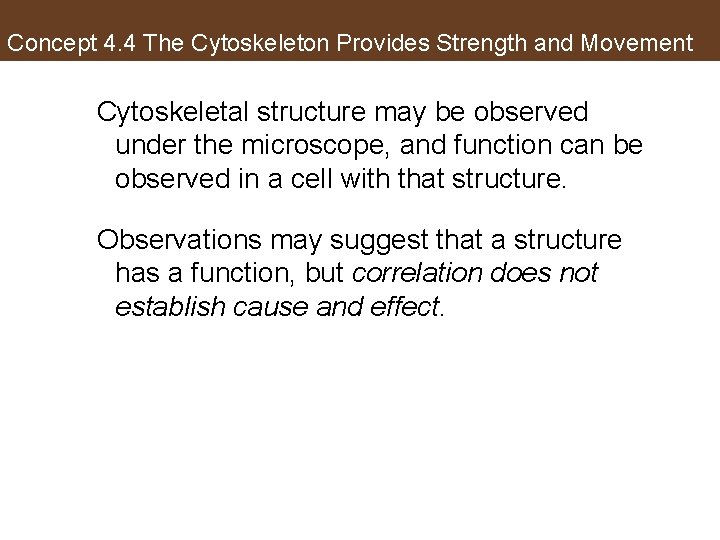
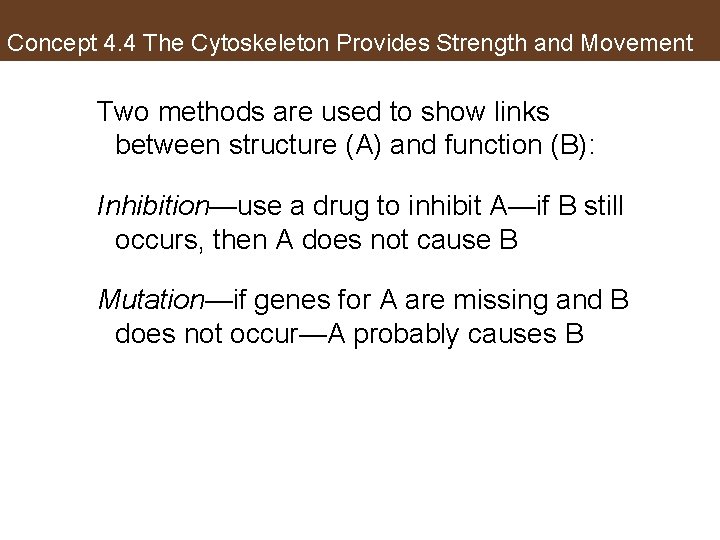
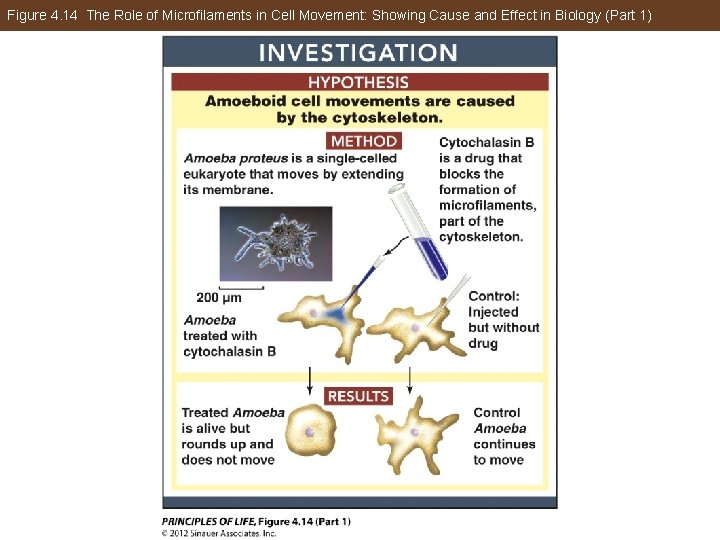
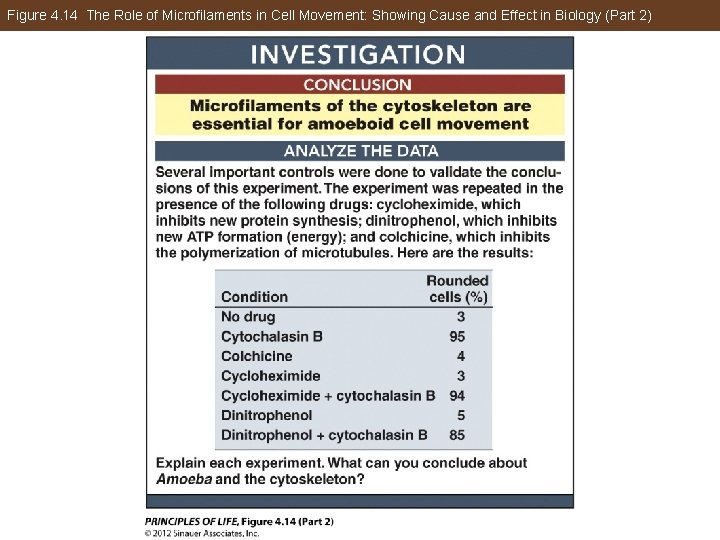
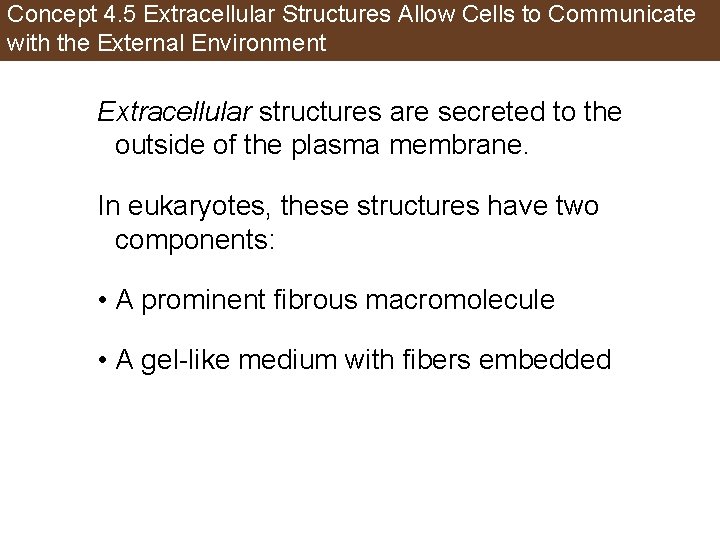
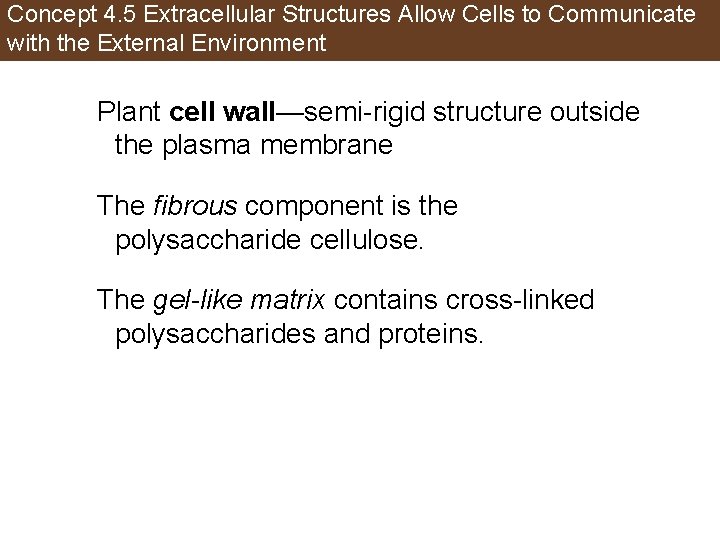
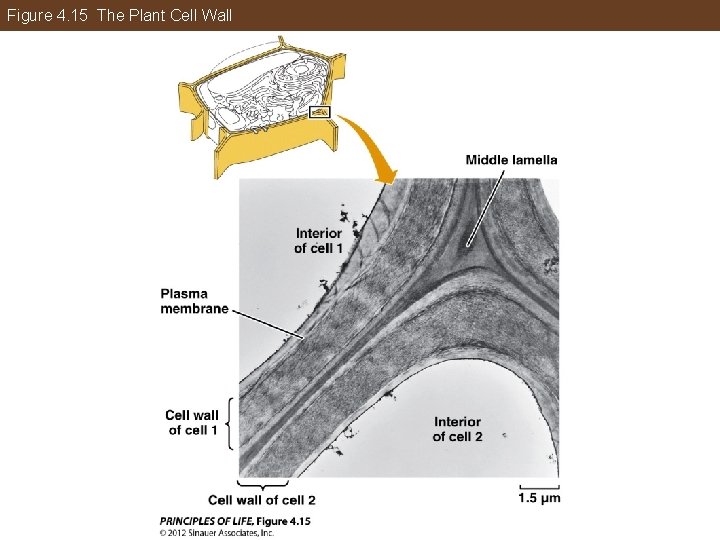
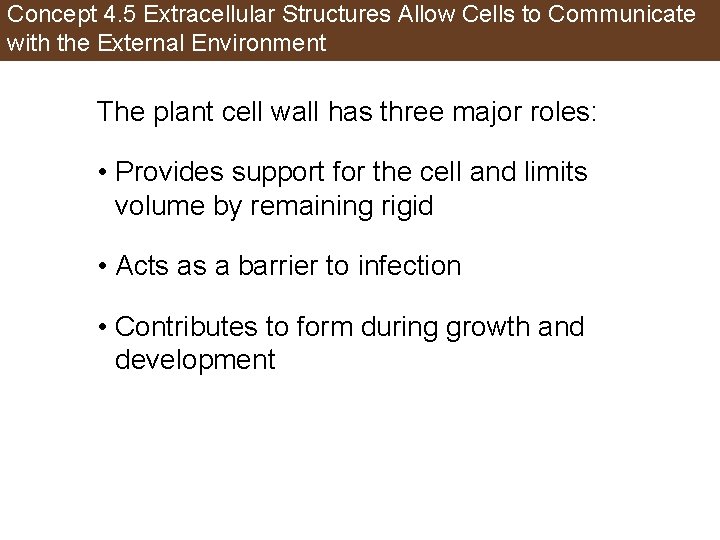
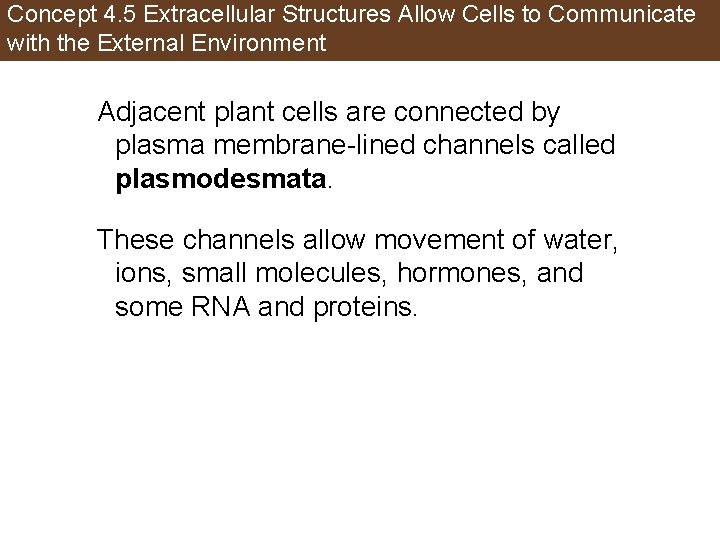
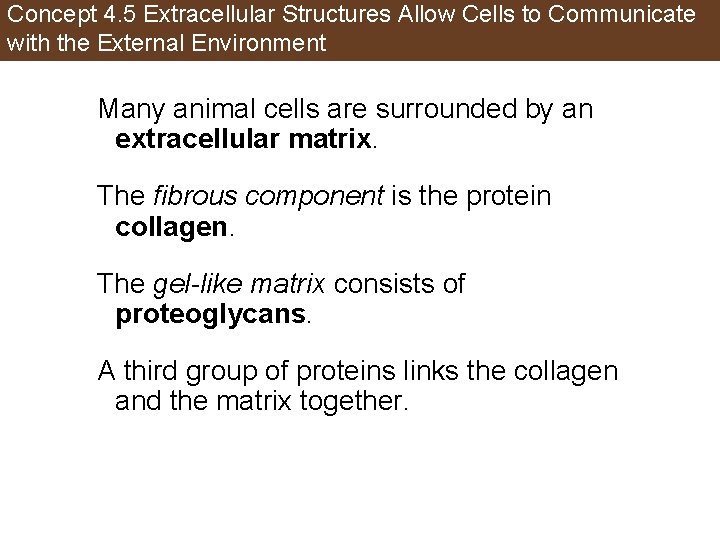
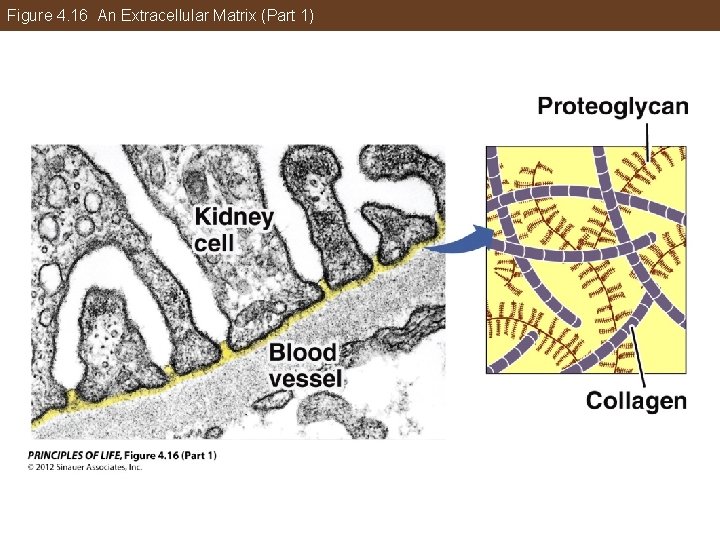
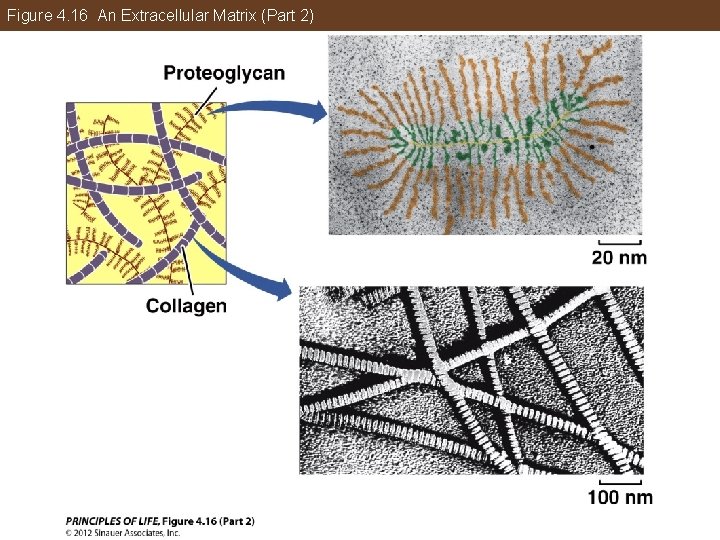
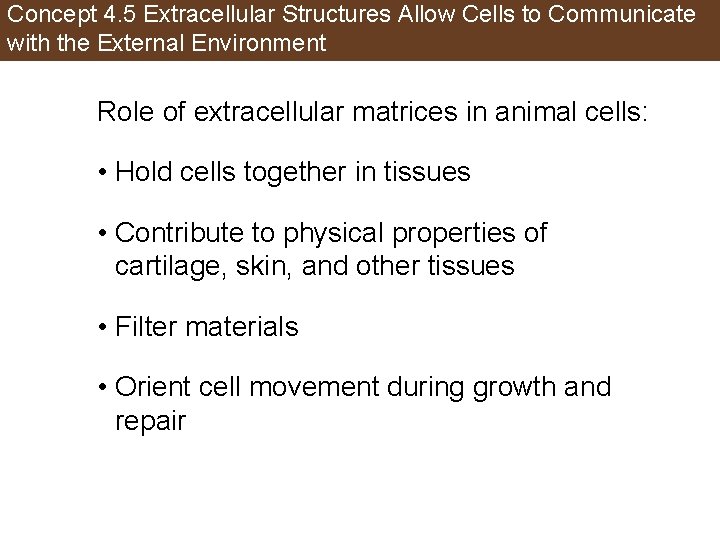
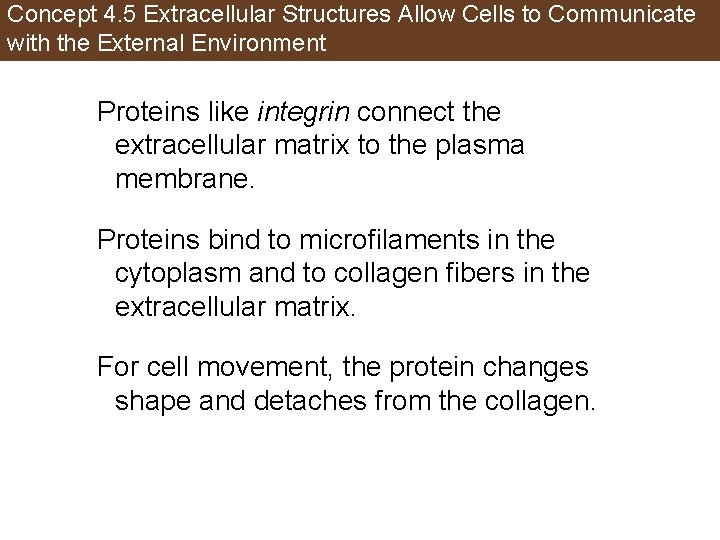
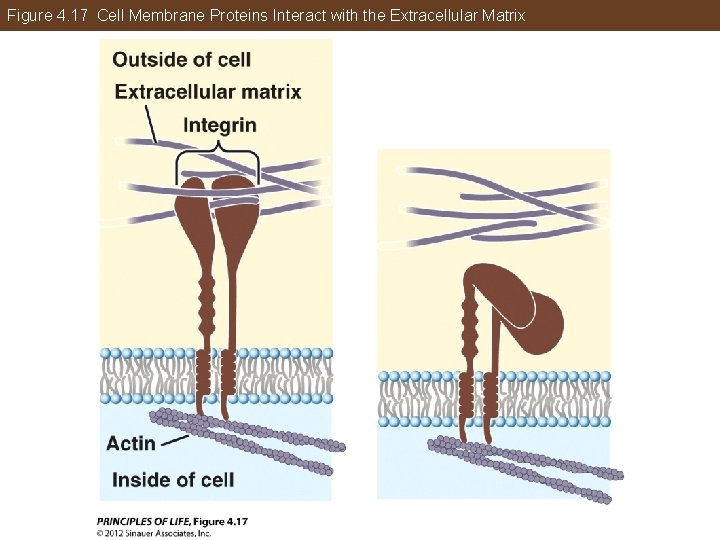
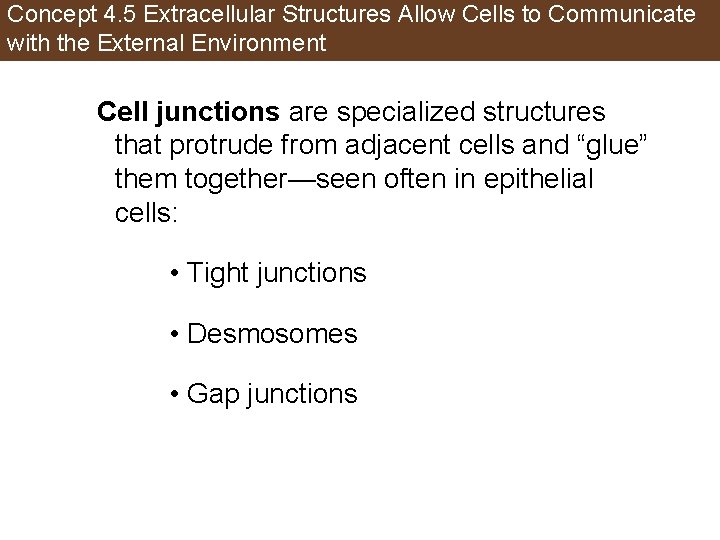
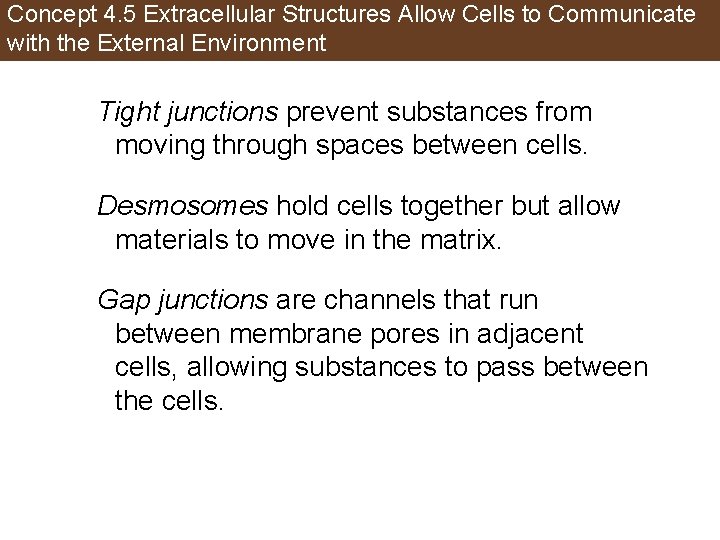
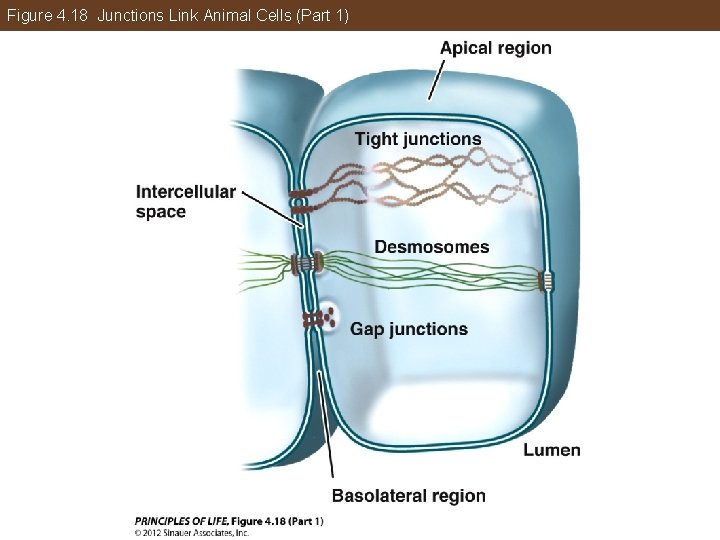
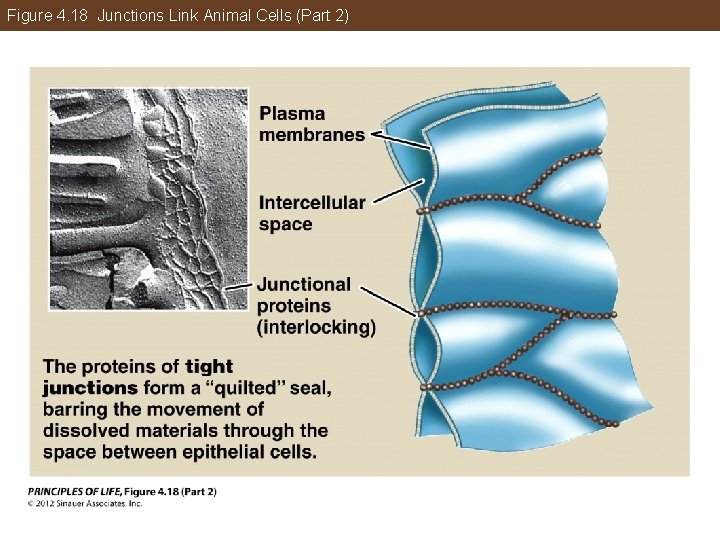
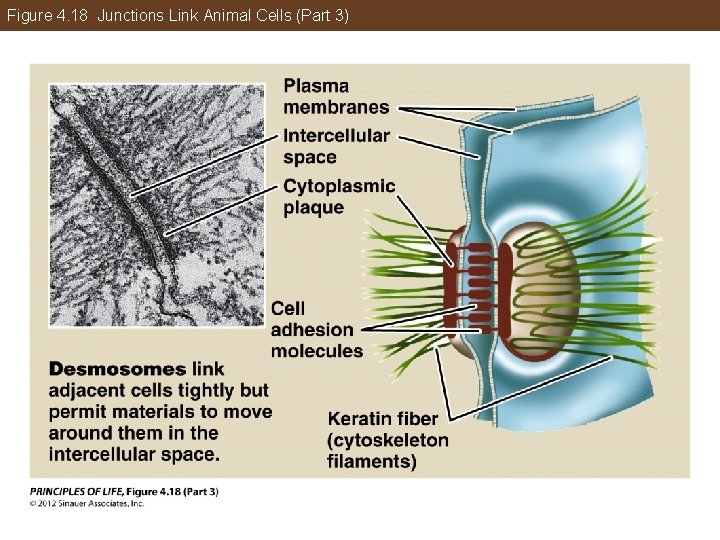
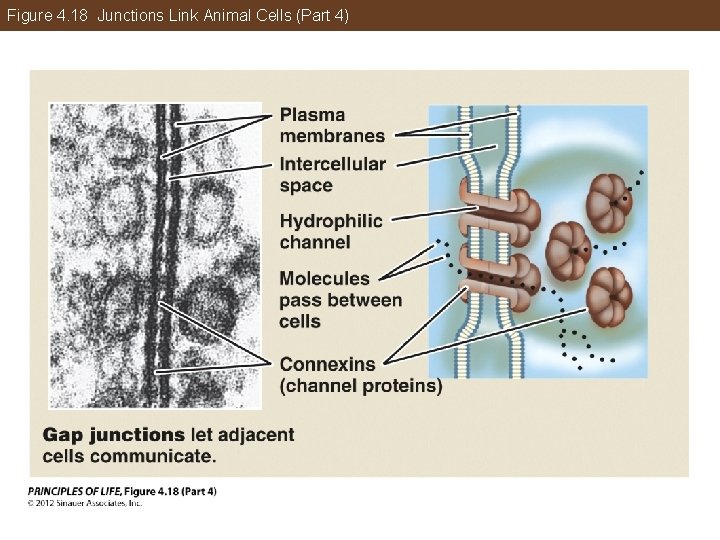
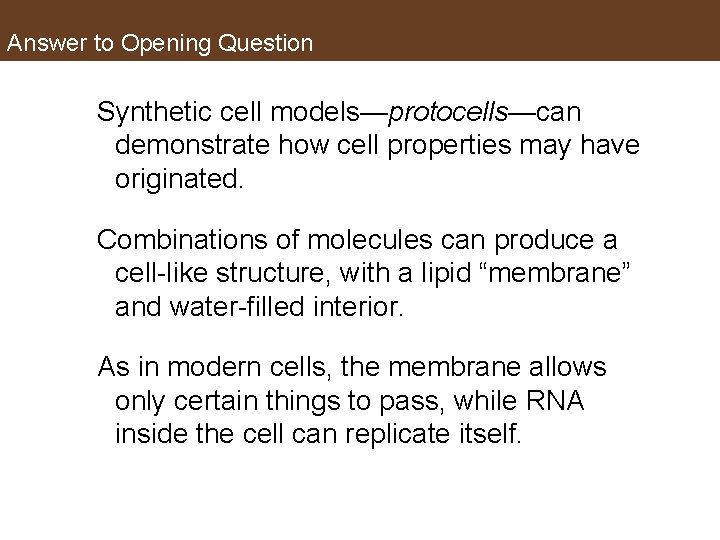
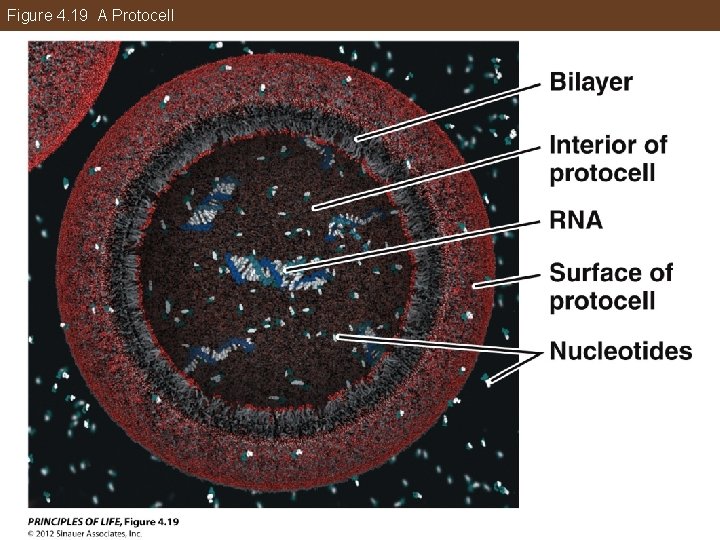
- Slides: 92
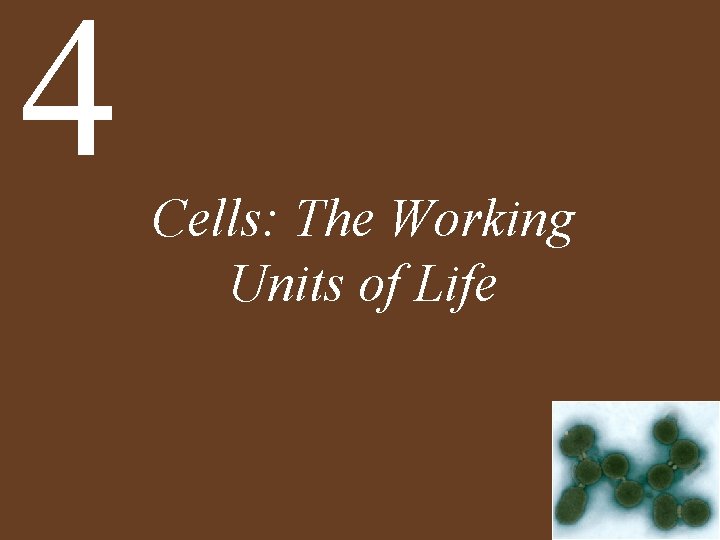
4 Cells: The Working Units of Life
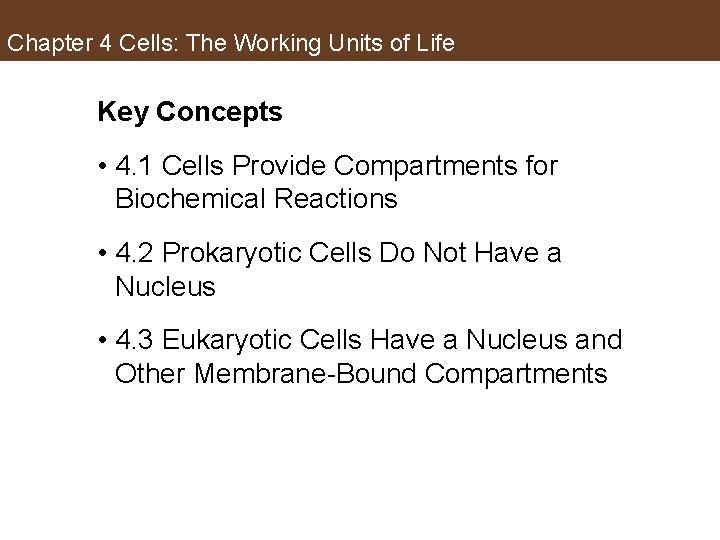
Chapter 4 Cells: The Working Units of Life Key Concepts • 4. 1 Cells Provide Compartments for Biochemical Reactions • 4. 2 Prokaryotic Cells Do Not Have a Nucleus • 4. 3 Eukaryotic Cells Have a Nucleus and Other Membrane-Bound Compartments
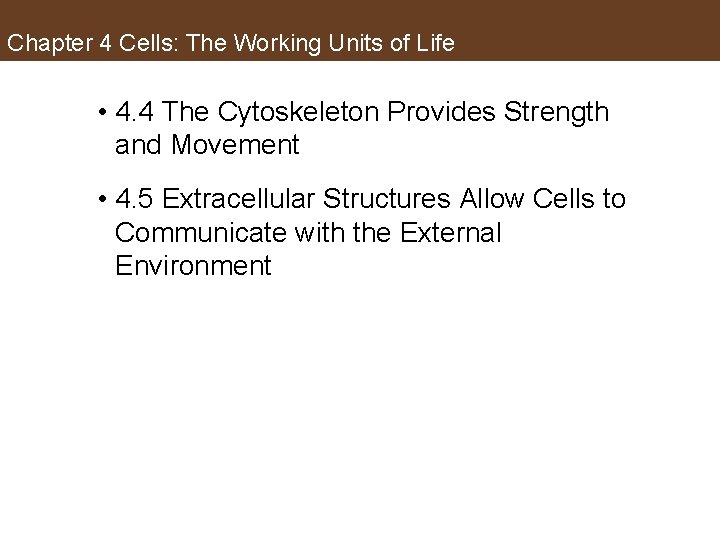
Chapter 4 Cells: The Working Units of Life • 4. 4 The Cytoskeleton Provides Strength and Movement • 4. 5 Extracellular Structures Allow Cells to Communicate with the External Environment
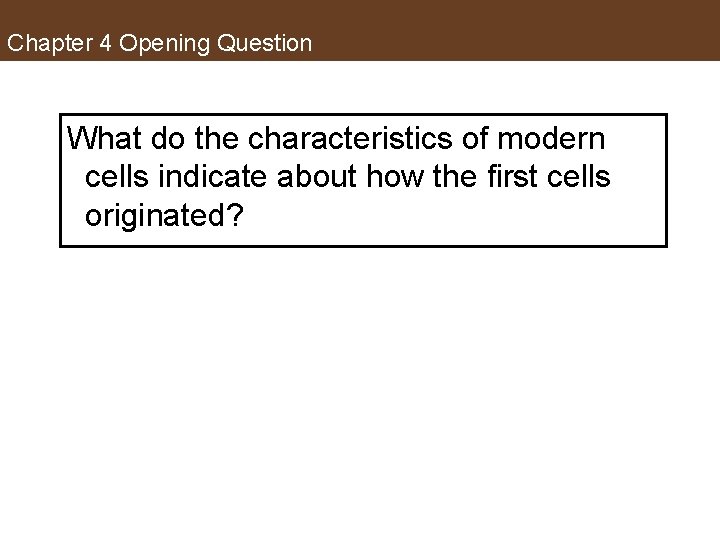
Chapter 4 Opening Question What do the characteristics of modern cells indicate about how the first cells originated?
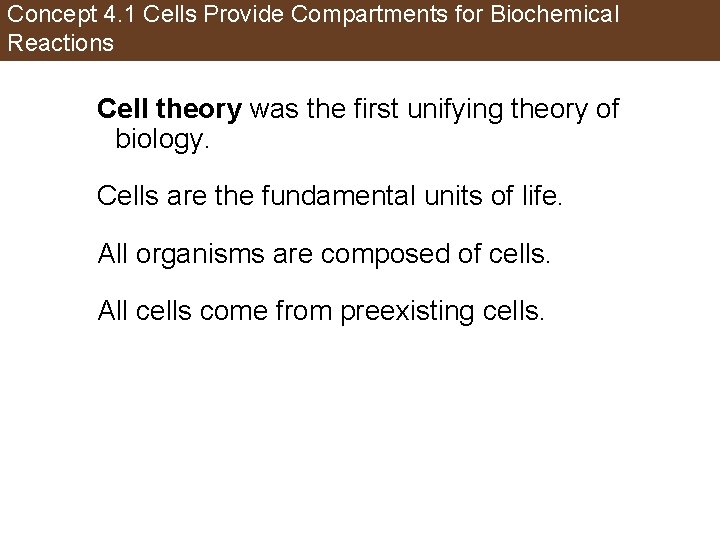
Concept 4. 1 Cells Provide Compartments for Biochemical Reactions Cell theory was the first unifying theory of biology. Cells are the fundamental units of life. All organisms are composed of cells. All cells come from preexisting cells.
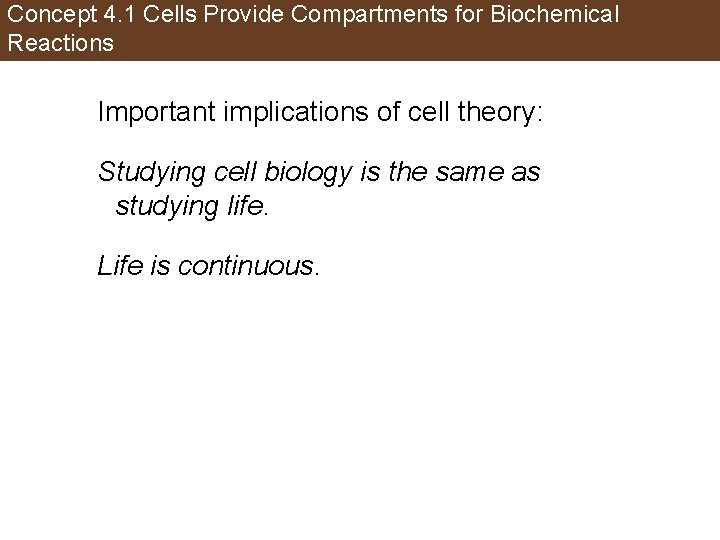
Concept 4. 1 Cells Provide Compartments for Biochemical Reactions Important implications of cell theory: Studying cell biology is the same as studying life. Life is continuous.
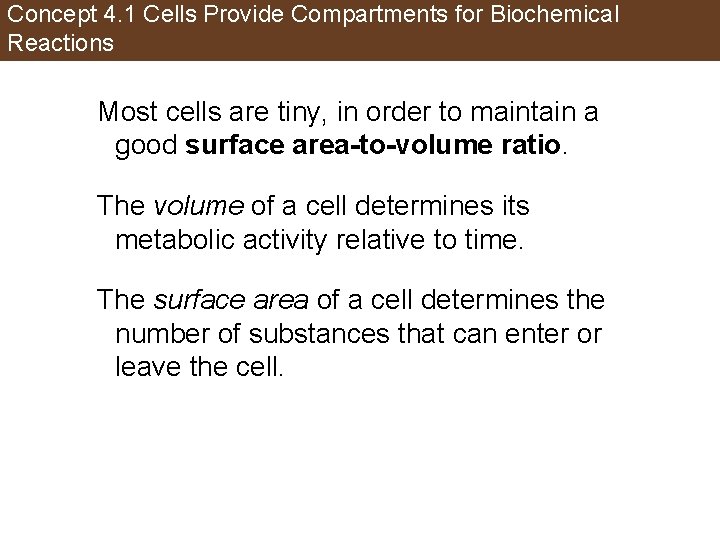
Concept 4. 1 Cells Provide Compartments for Biochemical Reactions Most cells are tiny, in order to maintain a good surface area-to-volume ratio. The volume of a cell determines its metabolic activity relative to time. The surface area of a cell determines the number of substances that can enter or leave the cell.
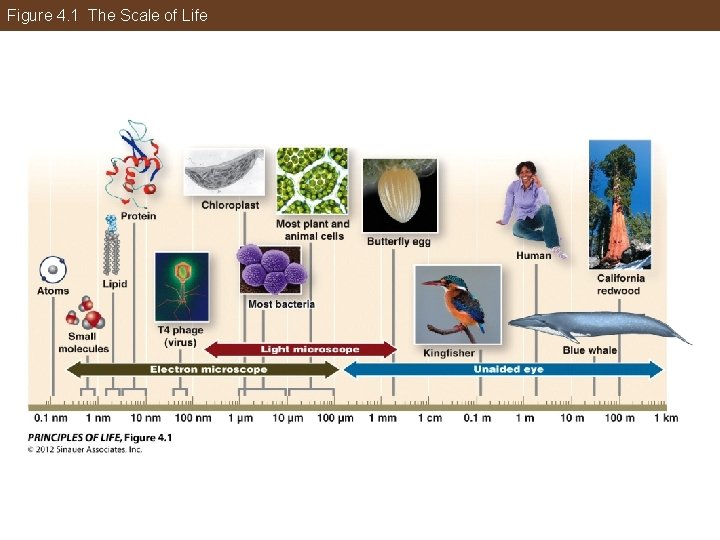
Figure 4. 1 The Scale of Life
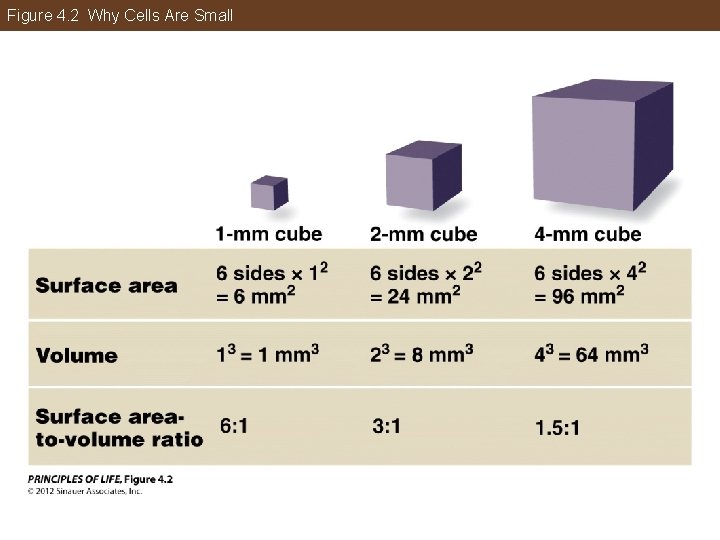
Figure 4. 2 Why Cells Are Small
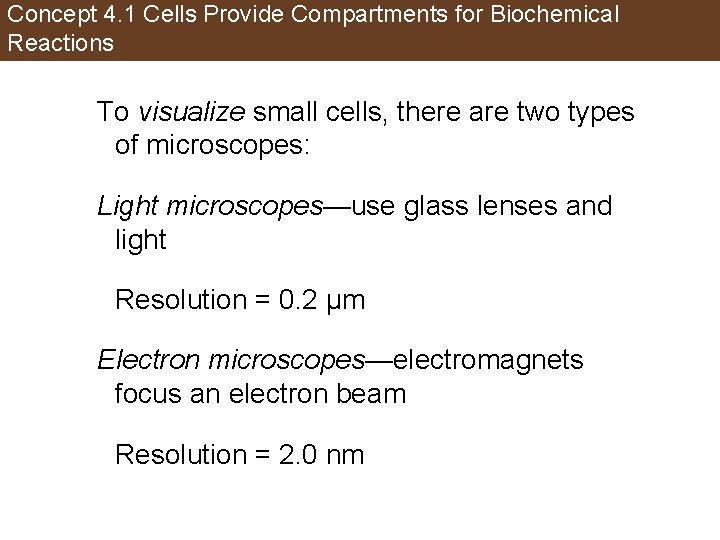
Concept 4. 1 Cells Provide Compartments for Biochemical Reactions To visualize small cells, there are two types of microscopes: Light microscopes—use glass lenses and light Resolution = 0. 2 μm Electron microscopes—electromagnets focus an electron beam Resolution = 2. 0 nm
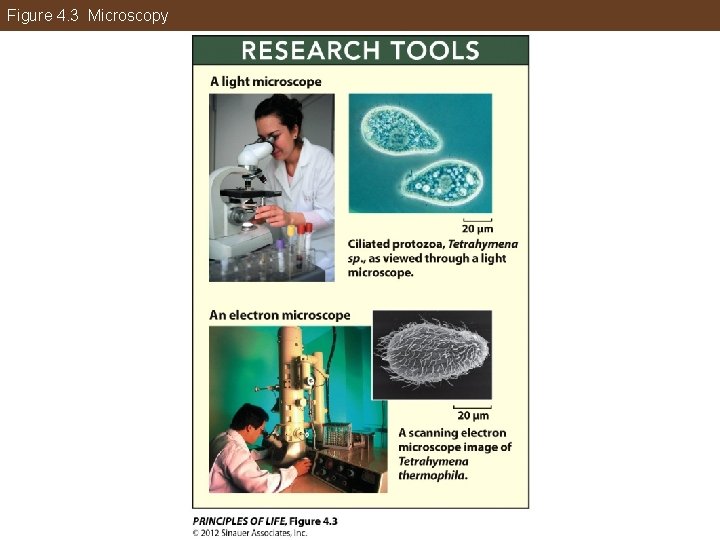
Figure 4. 3 Microscopy
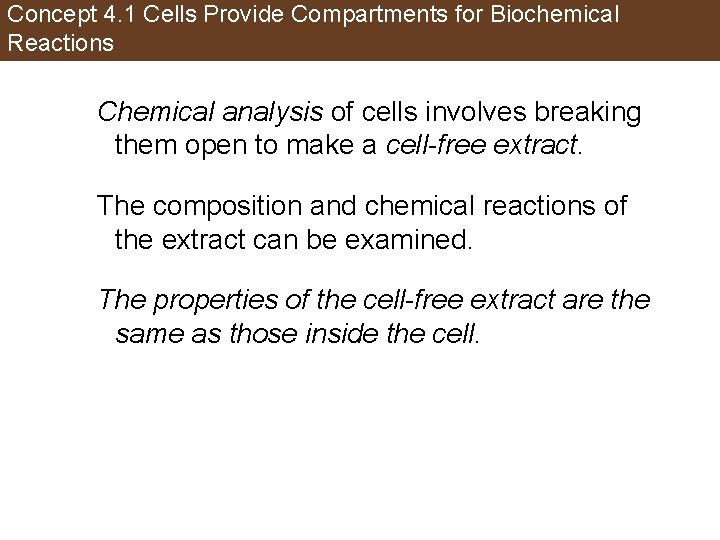
Concept 4. 1 Cells Provide Compartments for Biochemical Reactions Chemical analysis of cells involves breaking them open to make a cell-free extract. The composition and chemical reactions of the extract can be examined. The properties of the cell-free extract are the same as those inside the cell.
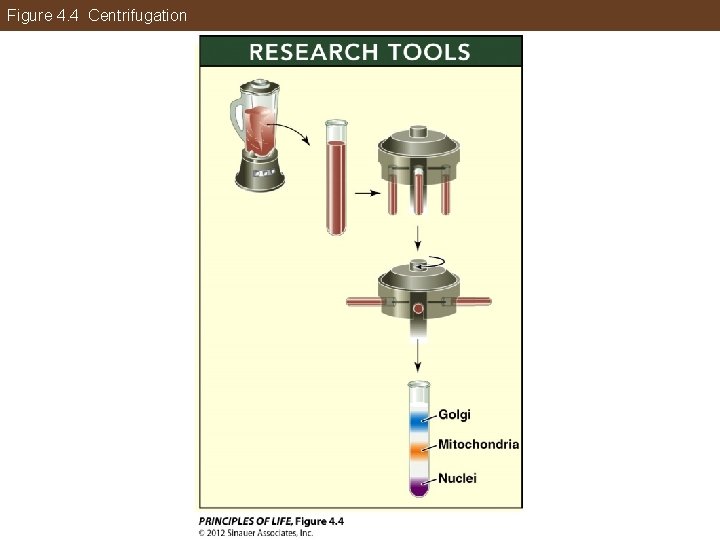
Figure 4. 4 Centrifugation
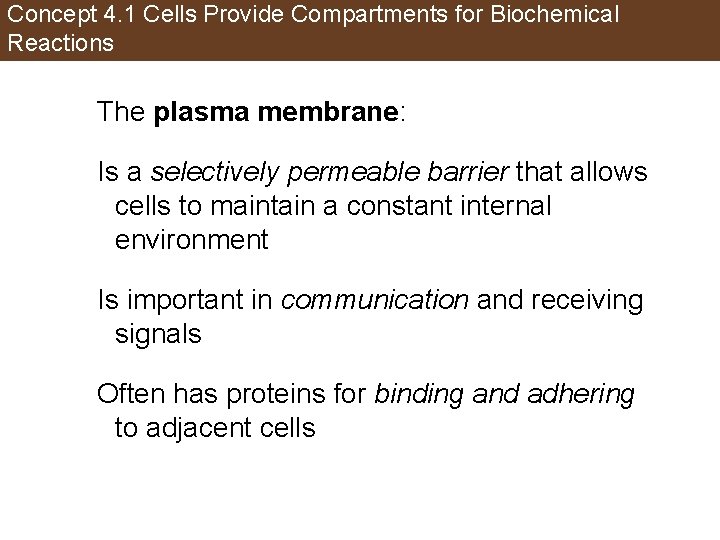
Concept 4. 1 Cells Provide Compartments for Biochemical Reactions The plasma membrane: Is a selectively permeable barrier that allows cells to maintain a constant internal environment Is important in communication and receiving signals Often has proteins for binding and adhering to adjacent cells
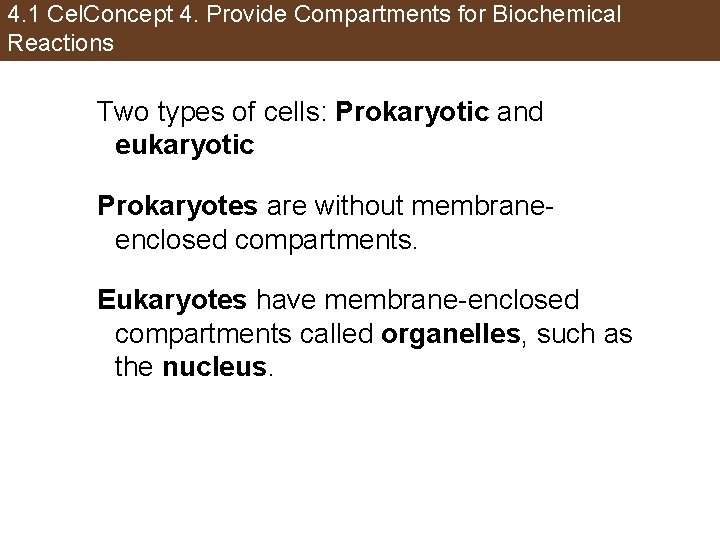
4. 1 Cel. Concept 4. Provide Compartments for Biochemical Reactions Two types of cells: Prokaryotic and eukaryotic Prokaryotes are without membraneenclosed compartments. Eukaryotes have membrane-enclosed compartments called organelles, such as the nucleus.
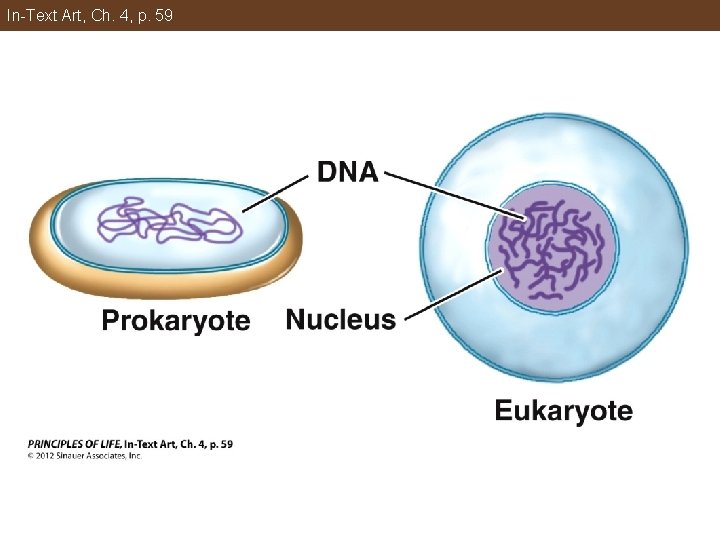
In-Text Art, Ch. 4, p. 59
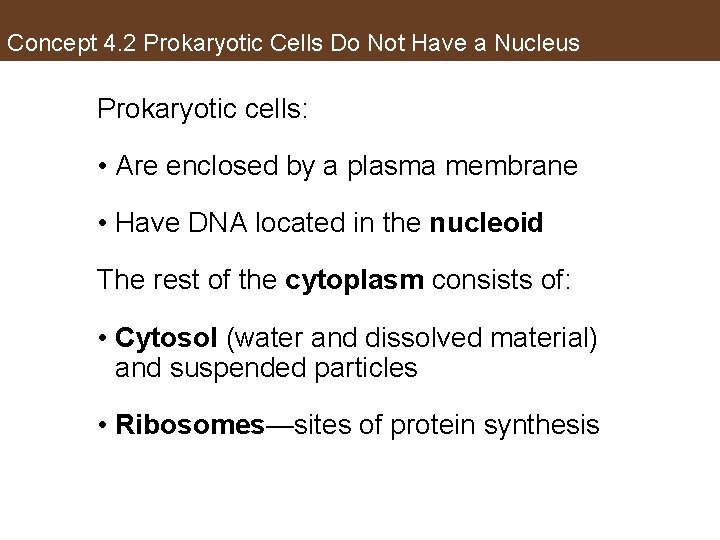
Concept 4. 2 Prokaryotic Cells Do Not Have a Nucleus Prokaryotic cells: • Are enclosed by a plasma membrane • Have DNA located in the nucleoid The rest of the cytoplasm consists of: • Cytosol (water and dissolved material) and suspended particles • Ribosomes—sites of protein synthesis
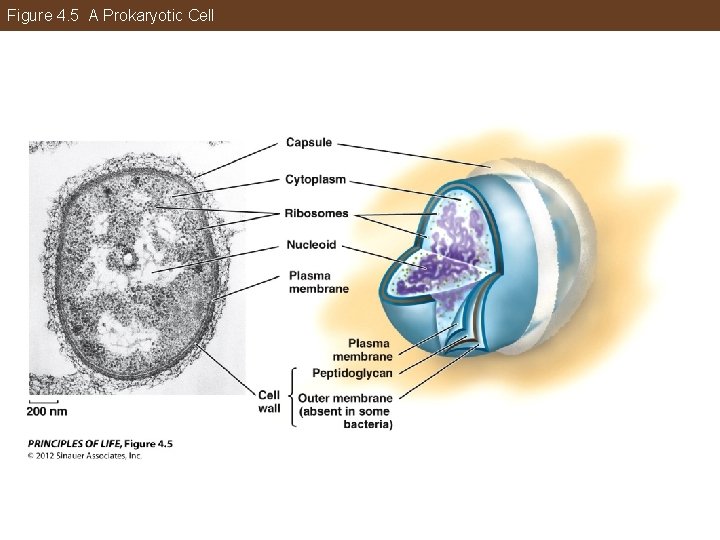
Figure 4. 5 A Prokaryotic Cell
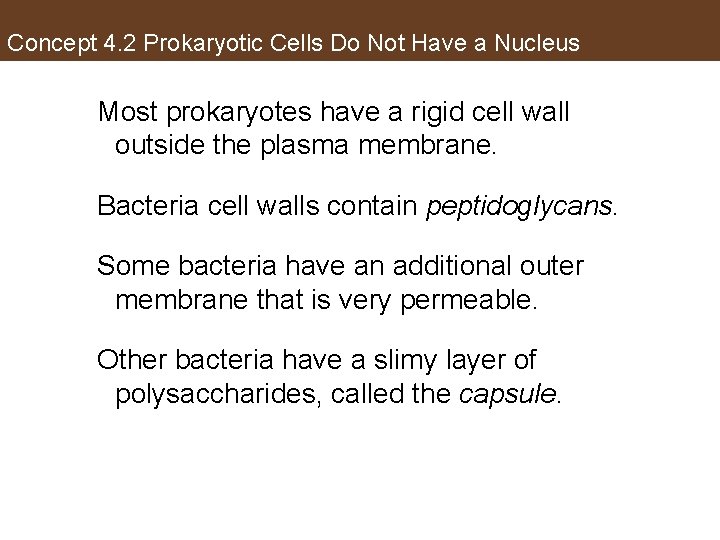
Concept 4. 2 Prokaryotic Cells Do Not Have a Nucleus Most prokaryotes have a rigid cell wall outside the plasma membrane. Bacteria cell walls contain peptidoglycans. Some bacteria have an additional outer membrane that is very permeable. Other bacteria have a slimy layer of polysaccharides, called the capsule.
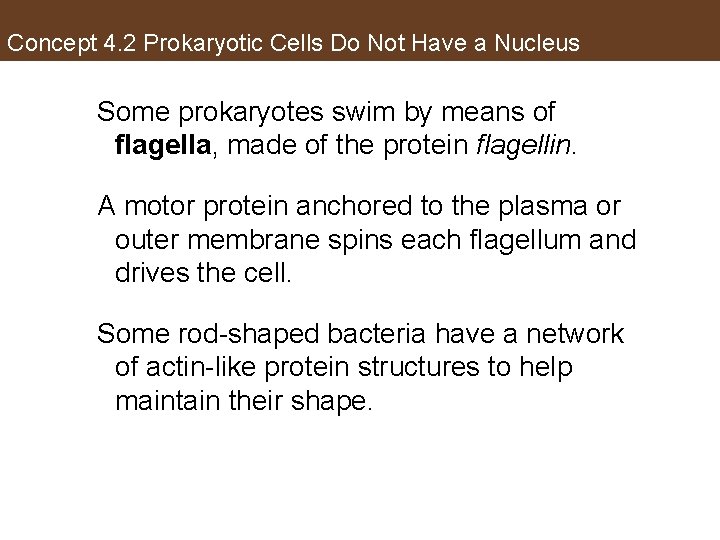
Concept 4. 2 Prokaryotic Cells Do Not Have a Nucleus Some prokaryotes swim by means of flagella, made of the protein flagellin. A motor protein anchored to the plasma or outer membrane spins each flagellum and drives the cell. Some rod-shaped bacteria have a network of actin-like protein structures to help maintain their shape.
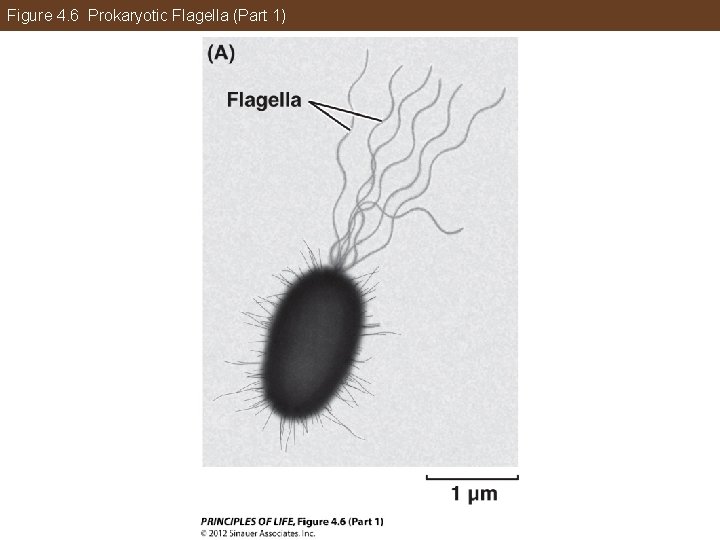
Figure 4. 6 Prokaryotic Flagella (Part 1)
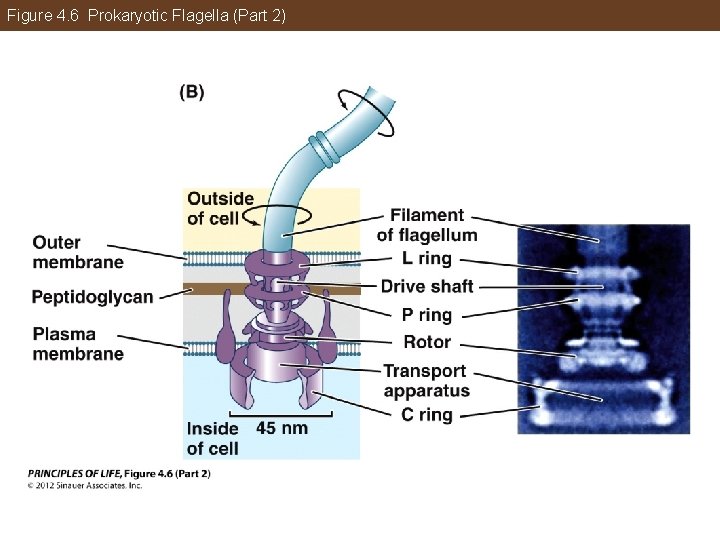
Figure 4. 6 Prokaryotic Flagella (Part 2)
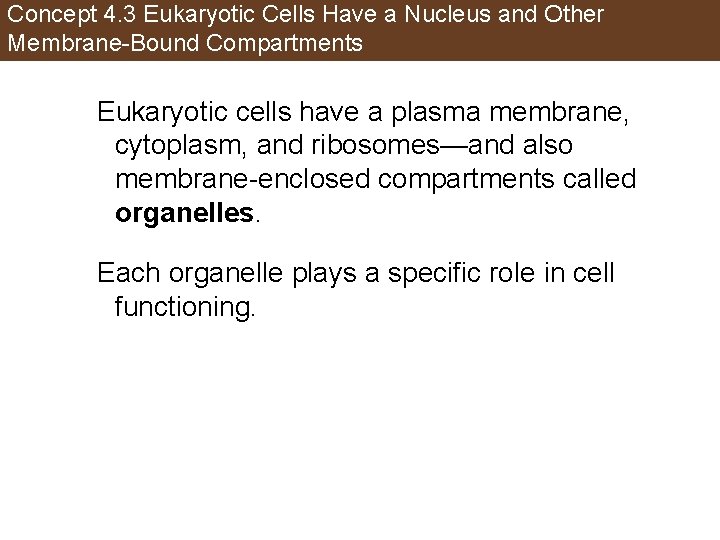
Concept 4. 3 Eukaryotic Cells Have a Nucleus and Other Membrane-Bound Compartments Eukaryotic cells have a plasma membrane, cytoplasm, and ribosomes—and also membrane-enclosed compartments called organelles. Each organelle plays a specific role in cell functioning.
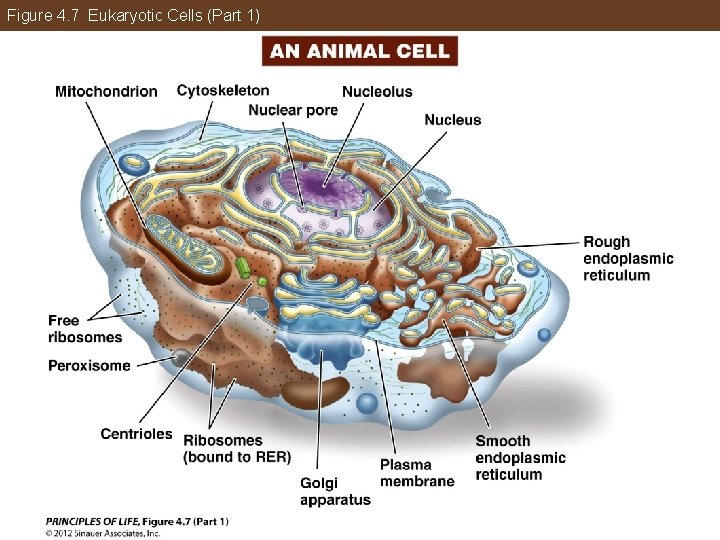
Figure 4. 7 Eukaryotic Cells (Part 1)
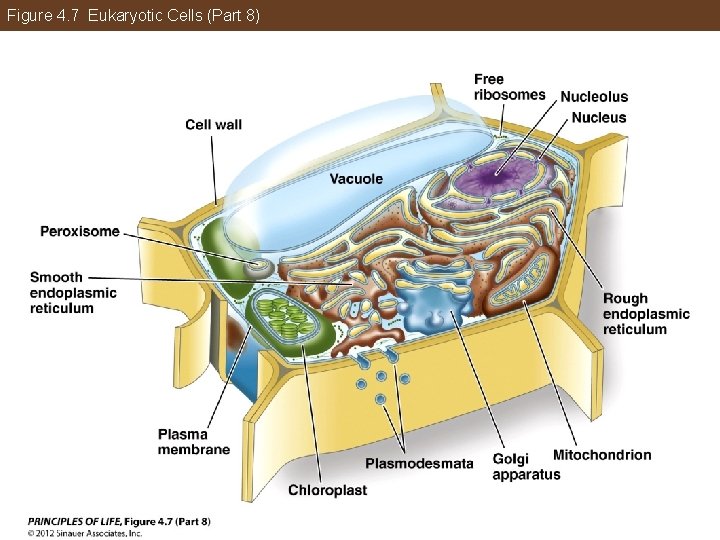
Figure 4. 7 Eukaryotic Cells (Part 8)
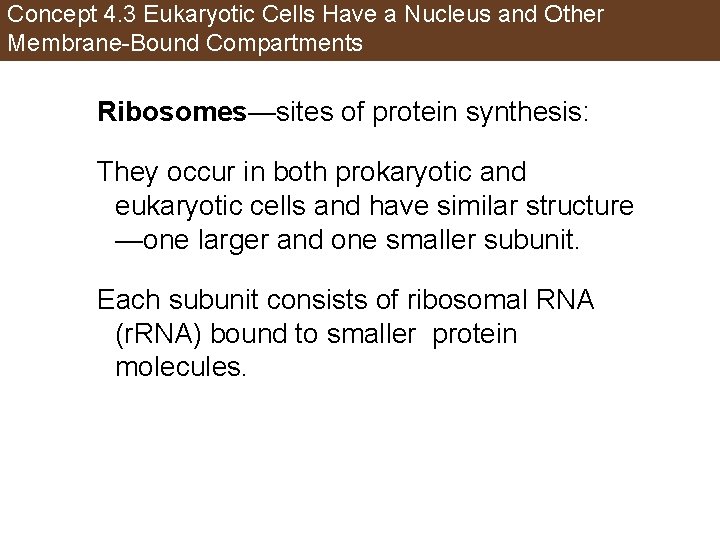
Concept 4. 3 Eukaryotic Cells Have a Nucleus and Other Membrane-Bound Compartments Ribosomes—sites of protein synthesis: They occur in both prokaryotic and eukaryotic cells and have similar structure —one larger and one smaller subunit. Each subunit consists of ribosomal RNA (r. RNA) bound to smaller protein molecules.
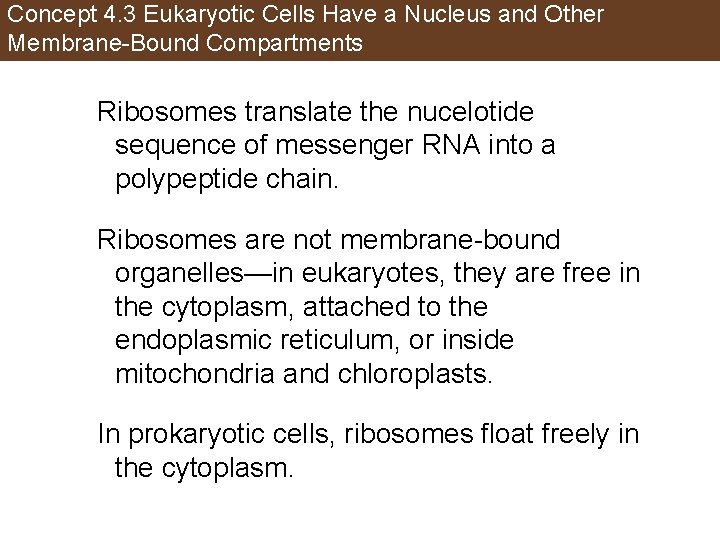
Concept 4. 3 Eukaryotic Cells Have a Nucleus and Other Membrane-Bound Compartments Ribosomes translate the nucelotide sequence of messenger RNA into a polypeptide chain. Ribosomes are not membrane-bound organelles—in eukaryotes, they are free in the cytoplasm, attached to the endoplasmic reticulum, or inside mitochondria and chloroplasts. In prokaryotic cells, ribosomes float freely in the cytoplasm.
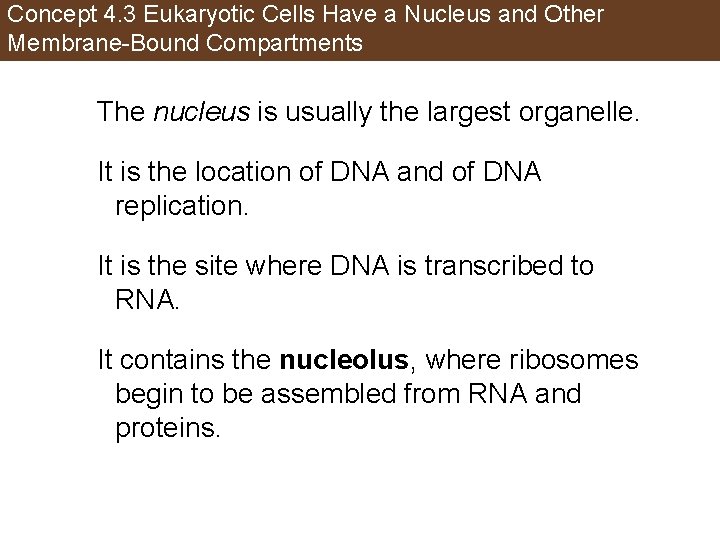
Concept 4. 3 Eukaryotic Cells Have a Nucleus and Other Membrane-Bound Compartments The nucleus is usually the largest organelle. It is the location of DNA and of DNA replication. It is the site where DNA is transcribed to RNA. It contains the nucleolus, where ribosomes begin to be assembled from RNA and proteins.
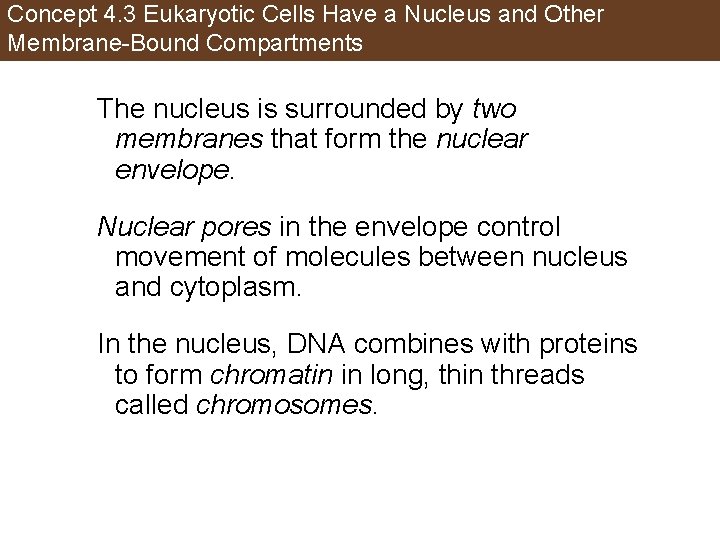
Concept 4. 3 Eukaryotic Cells Have a Nucleus and Other Membrane-Bound Compartments The nucleus is surrounded by two membranes that form the nuclear envelope. Nuclear pores in the envelope control movement of molecules between nucleus and cytoplasm. In the nucleus, DNA combines with proteins to form chromatin in long, thin threads called chromosomes.
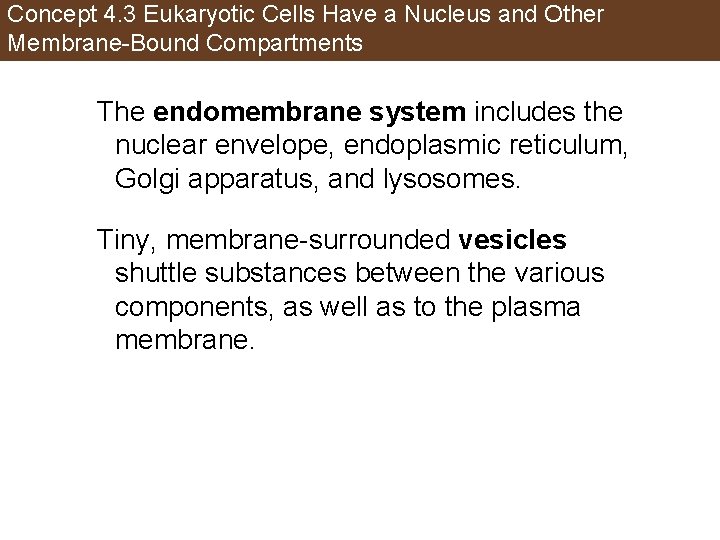
Concept 4. 3 Eukaryotic Cells Have a Nucleus and Other Membrane-Bound Compartments The endomembrane system includes the nuclear envelope, endoplasmic reticulum, Golgi apparatus, and lysosomes. Tiny, membrane-surrounded vesicles shuttle substances between the various components, as well as to the plasma membrane.
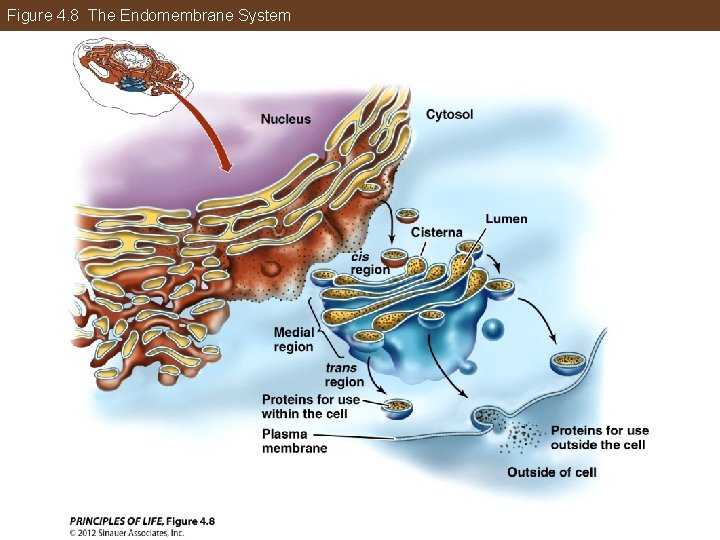
Figure 4. 8 The Endomembrane System
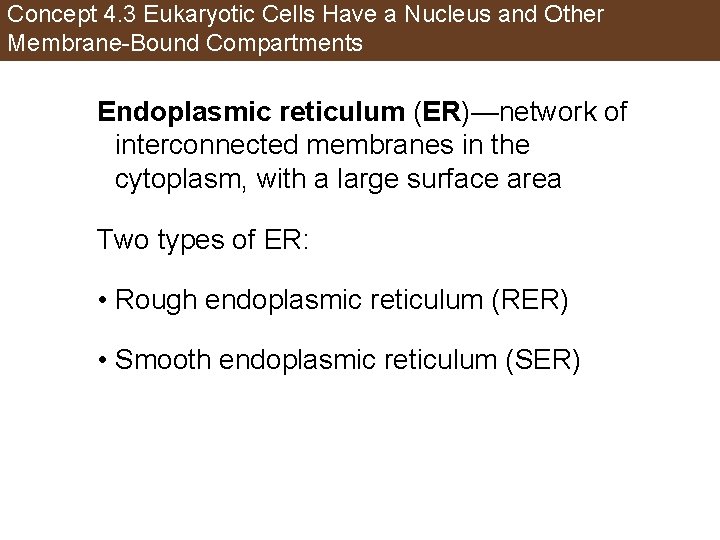
Concept 4. 3 Eukaryotic Cells Have a Nucleus and Other Membrane-Bound Compartments Endoplasmic reticulum (ER)—network of interconnected membranes in the cytoplasm, with a large surface area Two types of ER: • Rough endoplasmic reticulum (RER) • Smooth endoplasmic reticulum (SER)
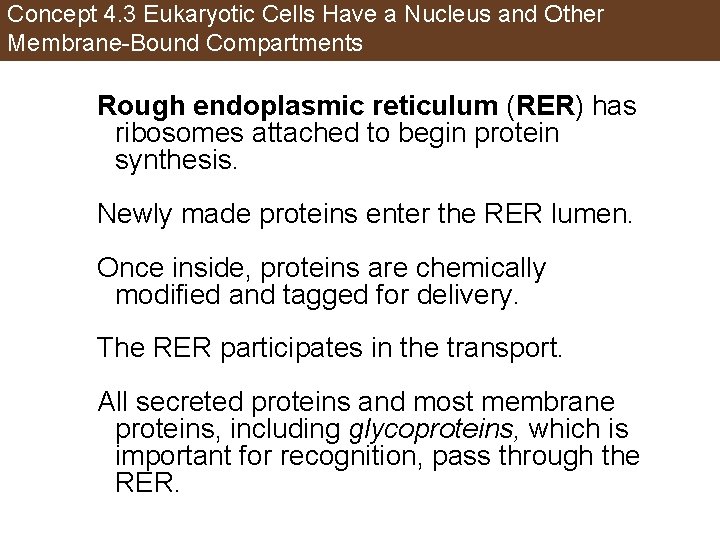
Concept 4. 3 Eukaryotic Cells Have a Nucleus and Other Membrane-Bound Compartments Rough endoplasmic reticulum (RER) has ribosomes attached to begin protein synthesis. Newly made proteins enter the RER lumen. Once inside, proteins are chemically modified and tagged for delivery. The RER participates in the transport. All secreted proteins and most membrane proteins, including glycoproteins, which is important for recognition, pass through the RER.
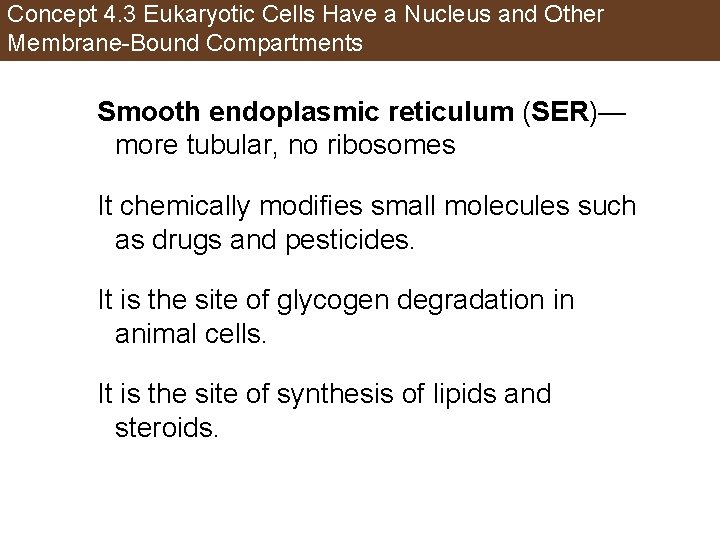
Concept 4. 3 Eukaryotic Cells Have a Nucleus and Other Membrane-Bound Compartments Smooth endoplasmic reticulum (SER)— more tubular, no ribosomes It chemically modifies small molecules such as drugs and pesticides. It is the site of glycogen degradation in animal cells. It is the site of synthesis of lipids and steroids.
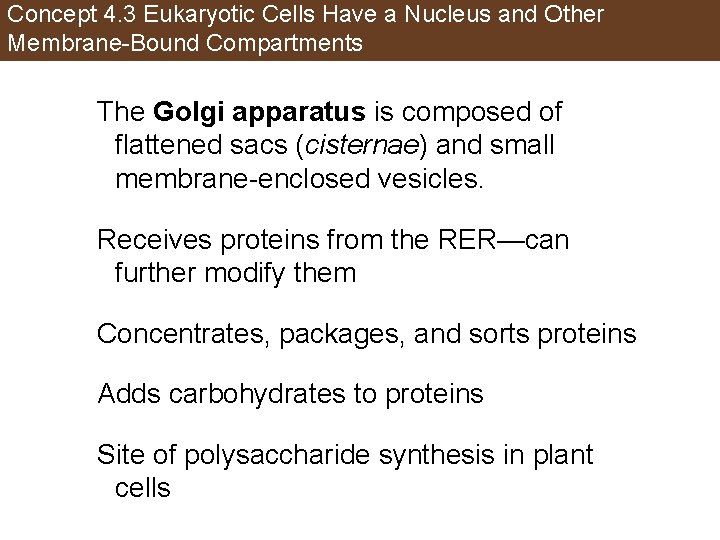
Concept 4. 3 Eukaryotic Cells Have a Nucleus and Other Membrane-Bound Compartments The Golgi apparatus is composed of flattened sacs (cisternae) and small membrane-enclosed vesicles. Receives proteins from the RER—can further modify them Concentrates, packages, and sorts proteins Adds carbohydrates to proteins Site of polysaccharide synthesis in plant cells
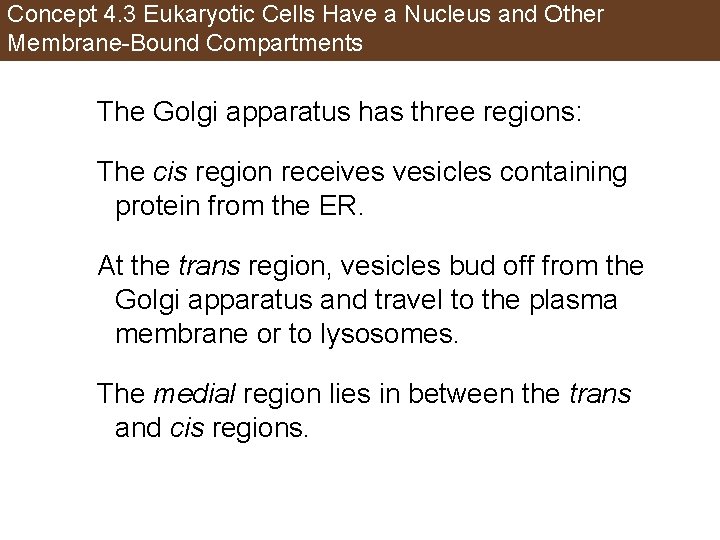
Concept 4. 3 Eukaryotic Cells Have a Nucleus and Other Membrane-Bound Compartments The Golgi apparatus has three regions: The cis region receives vesicles containing protein from the ER. At the trans region, vesicles bud off from the Golgi apparatus and travel to the plasma membrane or to lysosomes. The medial region lies in between the trans and cis regions.
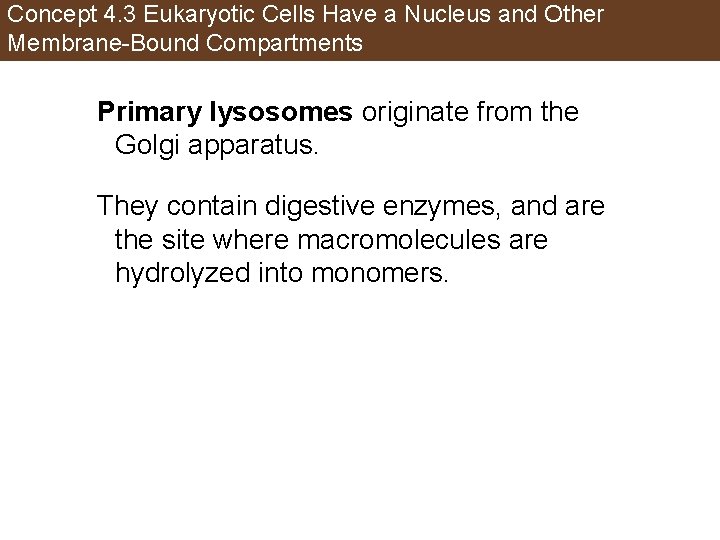
Concept 4. 3 Eukaryotic Cells Have a Nucleus and Other Membrane-Bound Compartments Primary lysosomes originate from the Golgi apparatus. They contain digestive enzymes, and are the site where macromolecules are hydrolyzed into monomers.
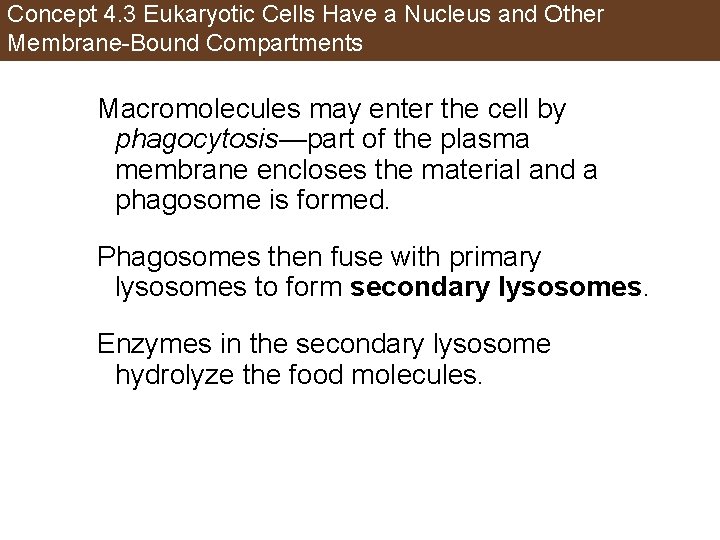
Concept 4. 3 Eukaryotic Cells Have a Nucleus and Other Membrane-Bound Compartments Macromolecules may enter the cell by phagocytosis—part of the plasma membrane encloses the material and a phagosome is formed. Phagosomes then fuse with primary lysosomes to form secondary lysosomes. Enzymes in the secondary lysosome hydrolyze the food molecules.
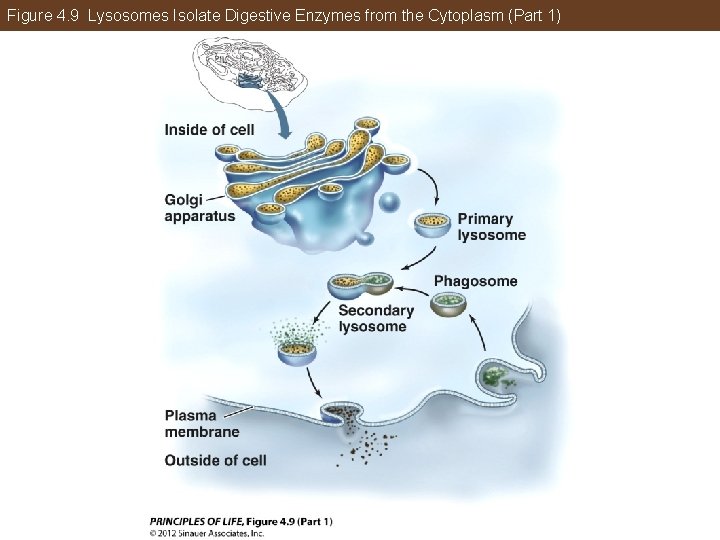
Figure 4. 9 Lysosomes Isolate Digestive Enzymes from the Cytoplasm (Part 1)
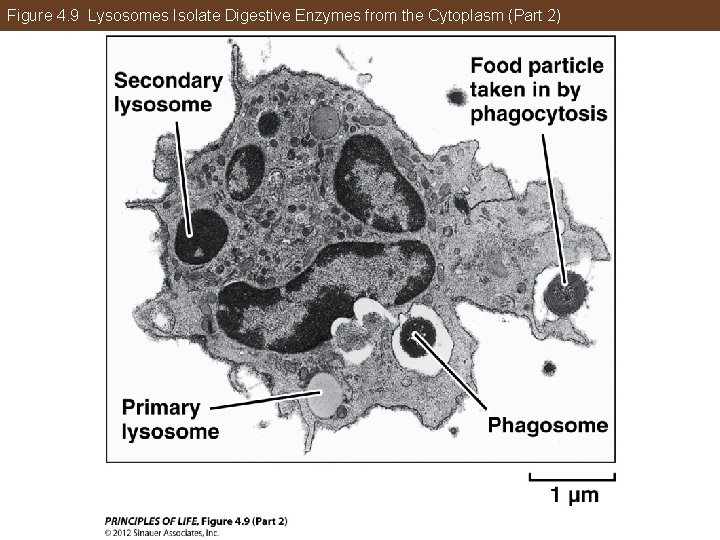
Figure 4. 9 Lysosomes Isolate Digestive Enzymes from the Cytoplasm (Part 2)
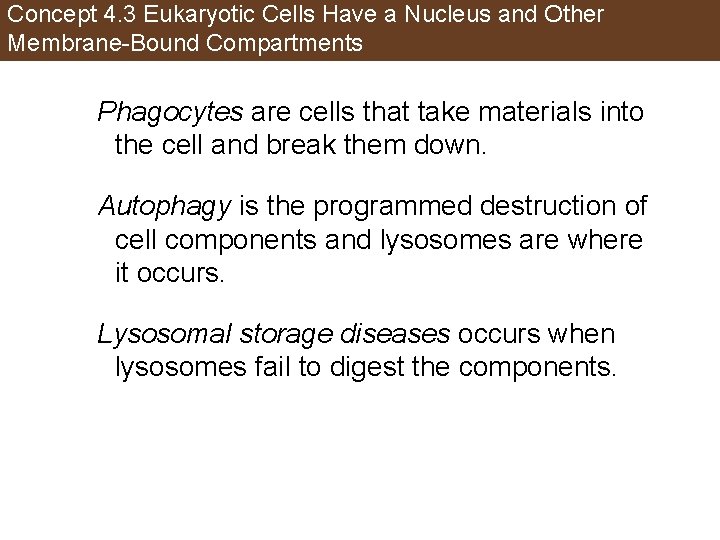
Concept 4. 3 Eukaryotic Cells Have a Nucleus and Other Membrane-Bound Compartments Phagocytes are cells that take materials into the cell and break them down. Autophagy is the programmed destruction of cell components and lysosomes are where it occurs. Lysosomal storage diseases occurs when lysosomes fail to digest the components.
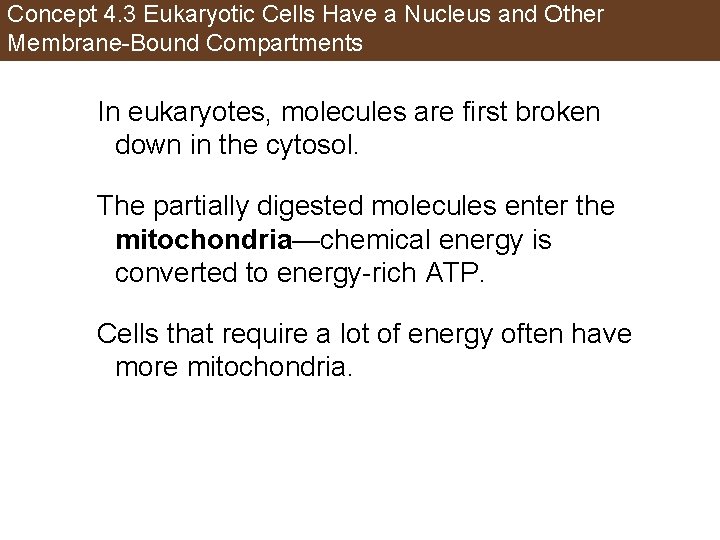
Concept 4. 3 Eukaryotic Cells Have a Nucleus and Other Membrane-Bound Compartments In eukaryotes, molecules are first broken down in the cytosol. The partially digested molecules enter the mitochondria—chemical energy is converted to energy-rich ATP. Cells that require a lot of energy often have more mitochondria.
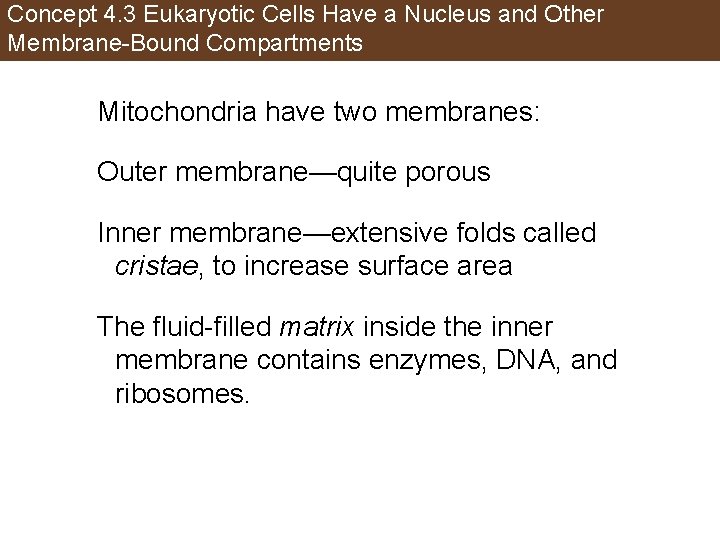
Concept 4. 3 Eukaryotic Cells Have a Nucleus and Other Membrane-Bound Compartments Mitochondria have two membranes: Outer membrane—quite porous Inner membrane—extensive folds called cristae, to increase surface area The fluid-filled matrix inside the inner membrane contains enzymes, DNA, and ribosomes.
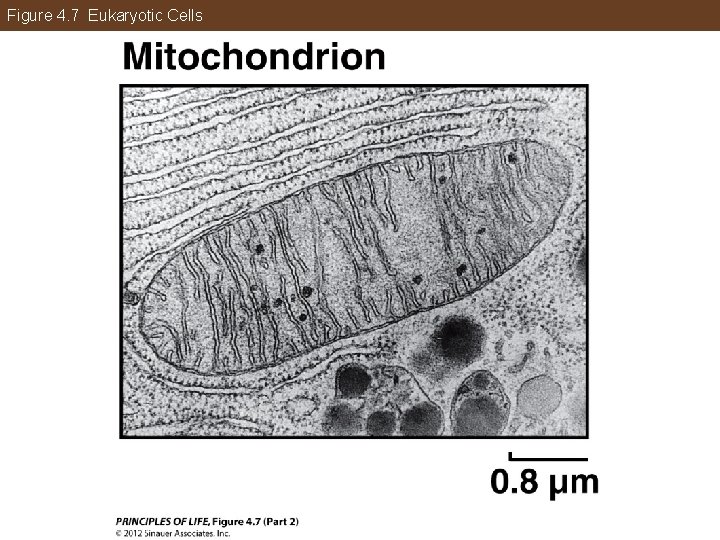
Figure 4. 7 Eukaryotic Cells
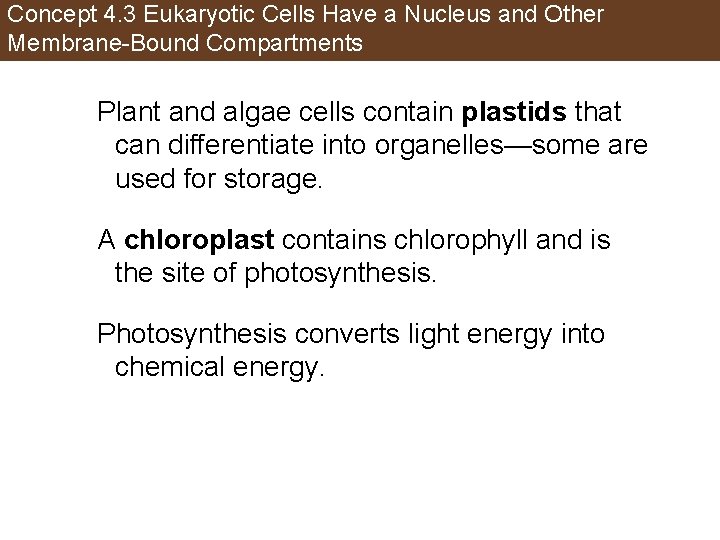
Concept 4. 3 Eukaryotic Cells Have a Nucleus and Other Membrane-Bound Compartments Plant and algae cells contain plastids that can differentiate into organelles—some are used for storage. A chloroplast contains chlorophyll and is the site of photosynthesis. Photosynthesis converts light energy into chemical energy.
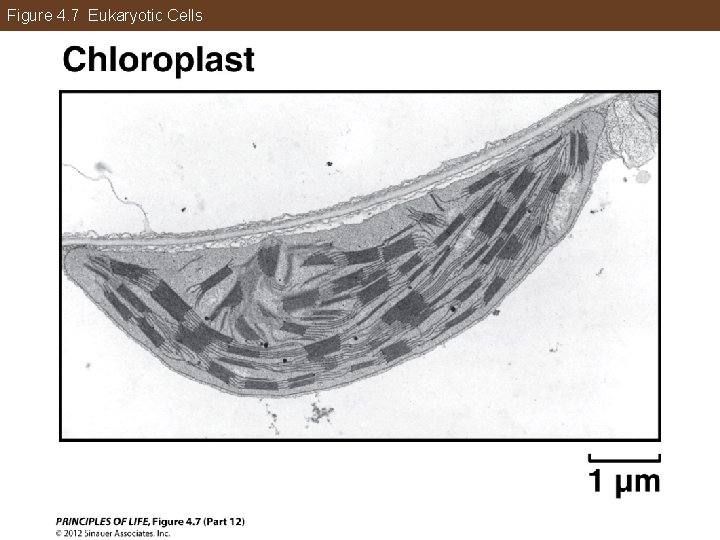
Figure 4. 7 Eukaryotic Cells
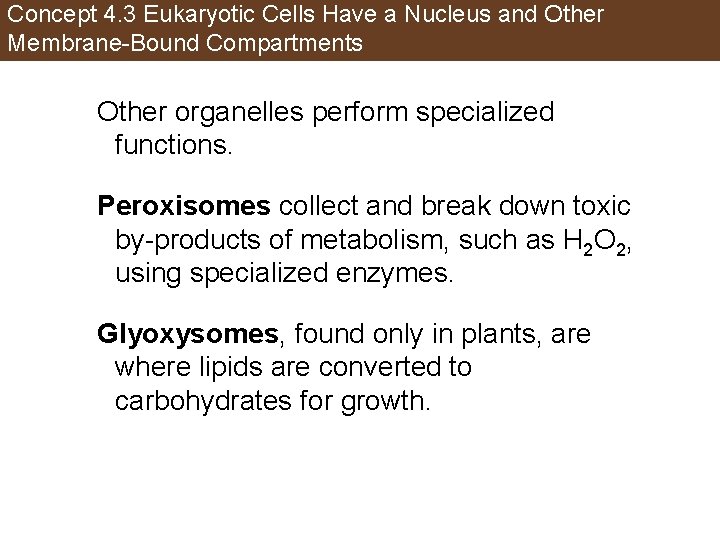
Concept 4. 3 Eukaryotic Cells Have a Nucleus and Other Membrane-Bound Compartments Other organelles perform specialized functions. Peroxisomes collect and break down toxic by-products of metabolism, such as H 2 O 2, using specialized enzymes. Glyoxysomes, found only in plants, are where lipids are converted to carbohydrates for growth.
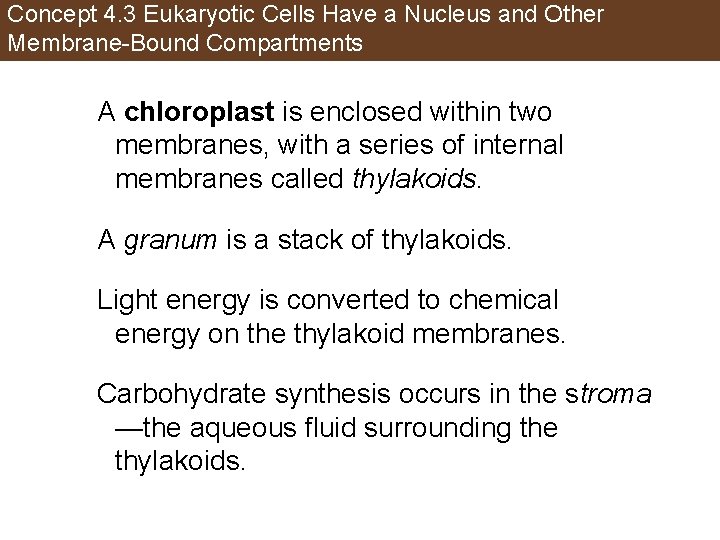
Concept 4. 3 Eukaryotic Cells Have a Nucleus and Other Membrane-Bound Compartments A chloroplast is enclosed within two membranes, with a series of internal membranes called thylakoids. A granum is a stack of thylakoids. Light energy is converted to chemical energy on the thylakoid membranes. Carbohydrate synthesis occurs in the stroma —the aqueous fluid surrounding the thylakoids.
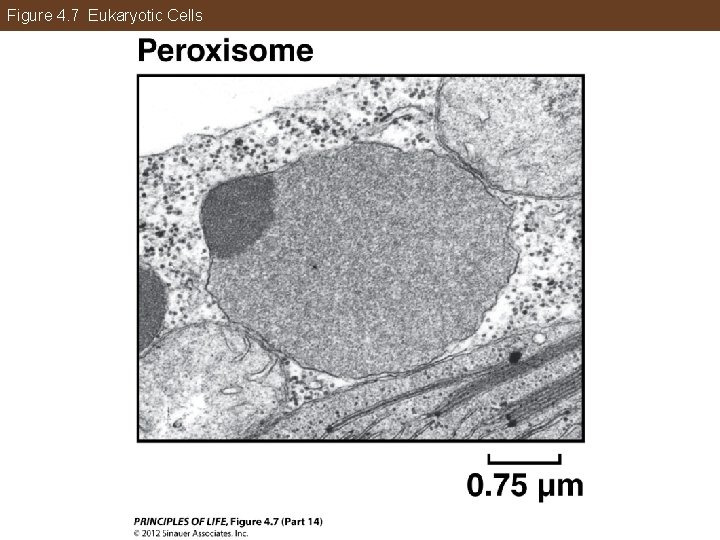
Figure 4. 7 Eukaryotic Cells
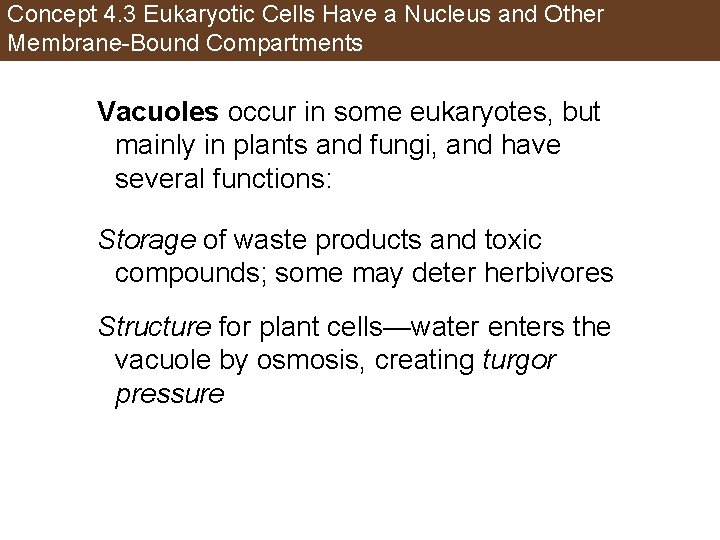
Concept 4. 3 Eukaryotic Cells Have a Nucleus and Other Membrane-Bound Compartments Vacuoles occur in some eukaryotes, but mainly in plants and fungi, and have several functions: Storage of waste products and toxic compounds; some may deter herbivores Structure for plant cells—water enters the vacuole by osmosis, creating turgor pressure
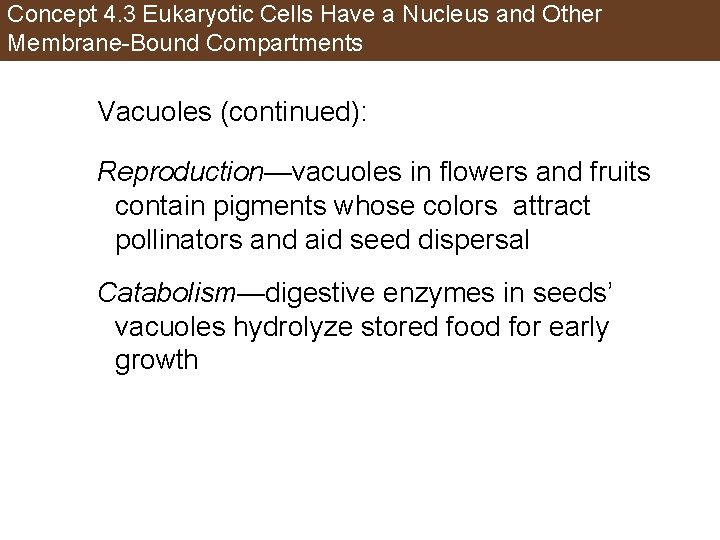
Concept 4. 3 Eukaryotic Cells Have a Nucleus and Other Membrane-Bound Compartments Vacuoles (continued): Reproduction—vacuoles in flowers and fruits contain pigments whose colors attract pollinators and aid seed dispersal Catabolism—digestive enzymes in seeds’ vacuoles hydrolyze stored food for early growth
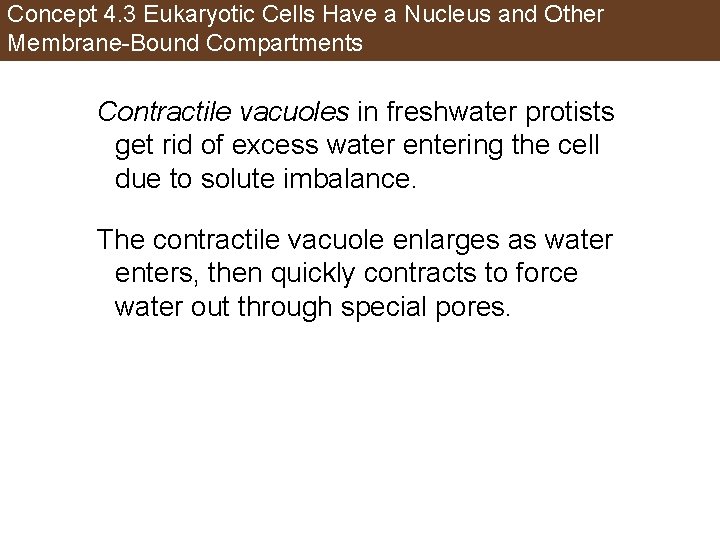
Concept 4. 3 Eukaryotic Cells Have a Nucleus and Other Membrane-Bound Compartments Contractile vacuoles in freshwater protists get rid of excess water entering the cell due to solute imbalance. The contractile vacuole enlarges as water enters, then quickly contracts to force water out through special pores.
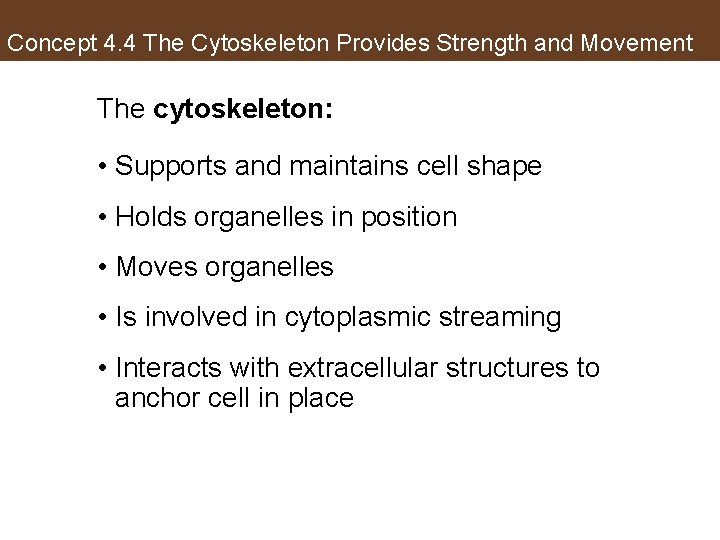
Concept 4. 4 The Cytoskeleton Provides Strength and Movement The cytoskeleton: • Supports and maintains cell shape • Holds organelles in position • Moves organelles • Is involved in cytoplasmic streaming • Interacts with extracellular structures to anchor cell in place
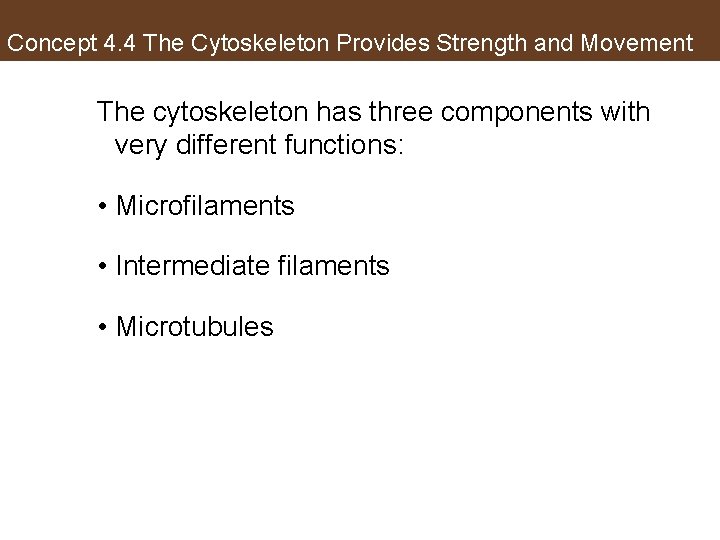
Concept 4. 4 The Cytoskeleton Provides Strength and Movement The cytoskeleton has three components with very different functions: • Microfilaments • Intermediate filaments • Microtubules
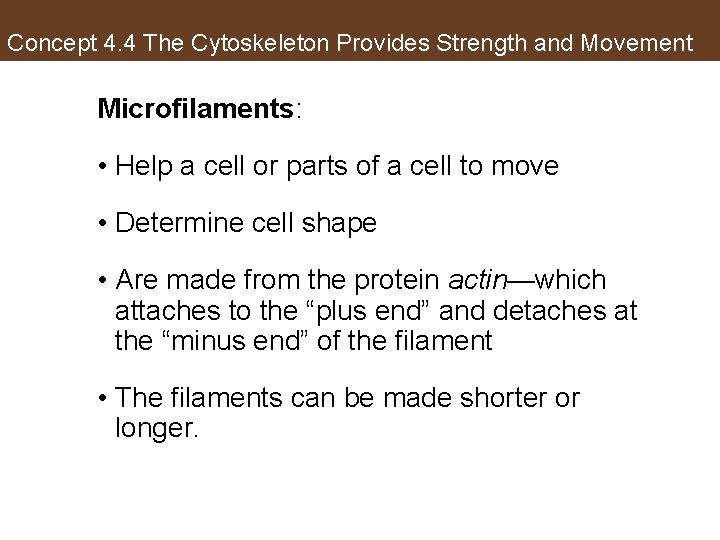
Concept 4. 4 The Cytoskeleton Provides Strength and Movement Microfilaments: • Help a cell or parts of a cell to move • Determine cell shape • Are made from the protein actin—which attaches to the “plus end” and detaches at the “minus end” of the filament • The filaments can be made shorter or longer.
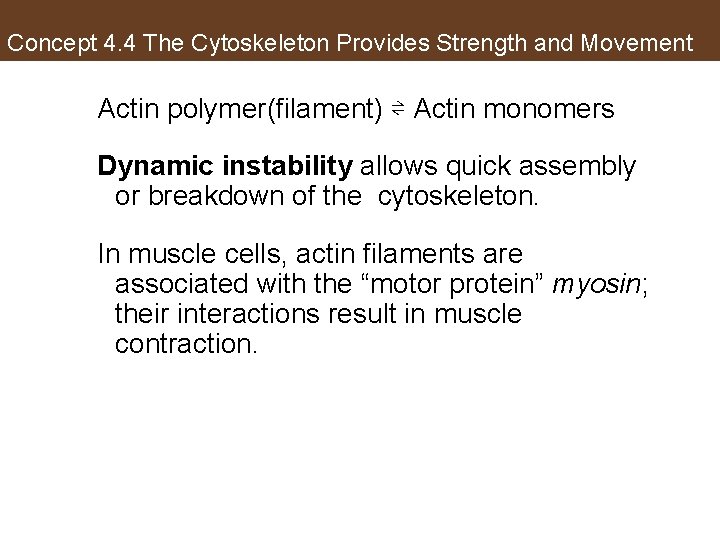
Concept 4. 4 The Cytoskeleton Provides Strength and Movement Actin polymer(filament) ⇌ Actin monomers Dynamic instability allows quick assembly or breakdown of the cytoskeleton. In muscle cells, actin filaments are associated with the “motor protein” myosin; their interactions result in muscle contraction.
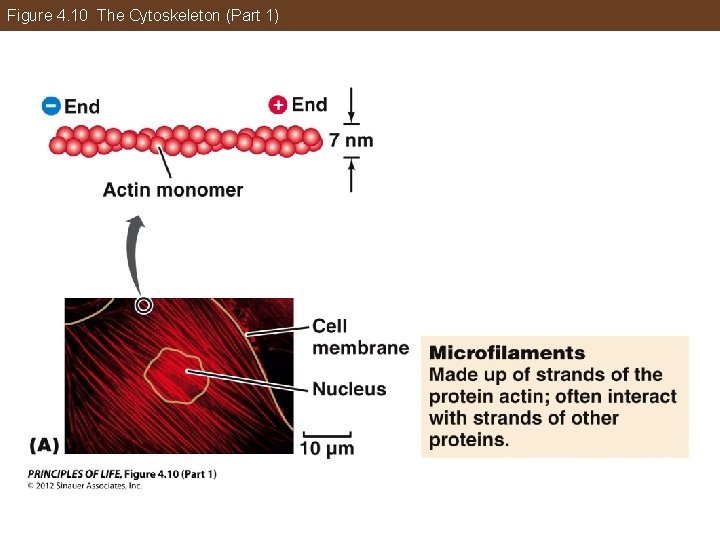
Figure 4. 10 The Cytoskeleton (Part 1)
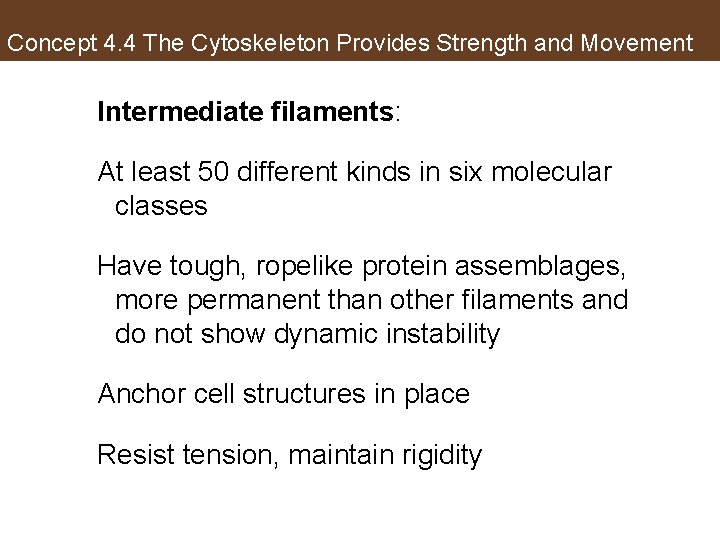
Concept 4. 4 The Cytoskeleton Provides Strength and Movement Intermediate filaments: At least 50 different kinds in six molecular classes Have tough, ropelike protein assemblages, more permanent than other filaments and do not show dynamic instability Anchor cell structures in place Resist tension, maintain rigidity
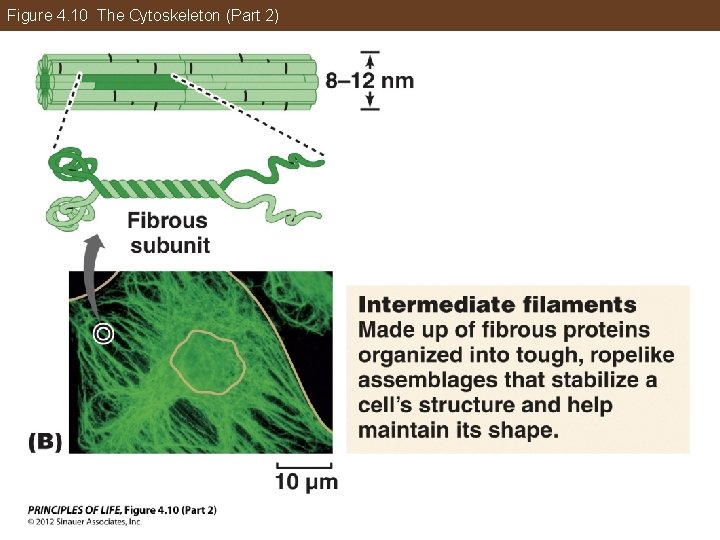
Figure 4. 10 The Cytoskeleton (Part 2)
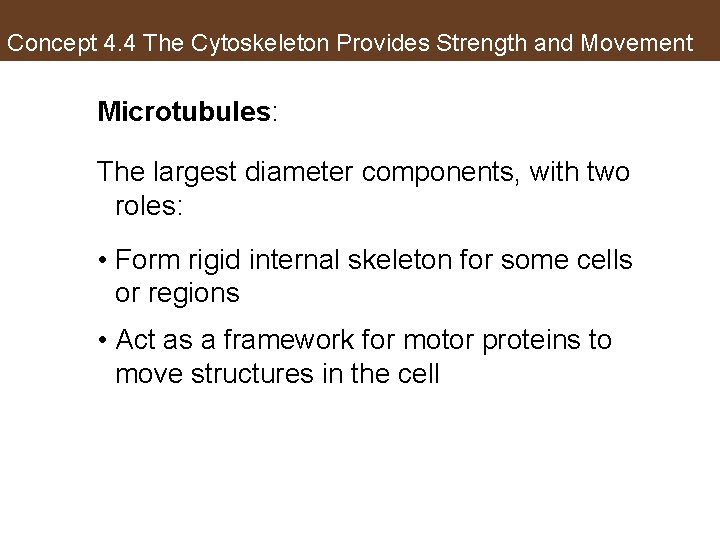
Concept 4. 4 The Cytoskeleton Provides Strength and Movement Microtubules: The largest diameter components, with two roles: • Form rigid internal skeleton for some cells or regions • Act as a framework for motor proteins to move structures in the cell
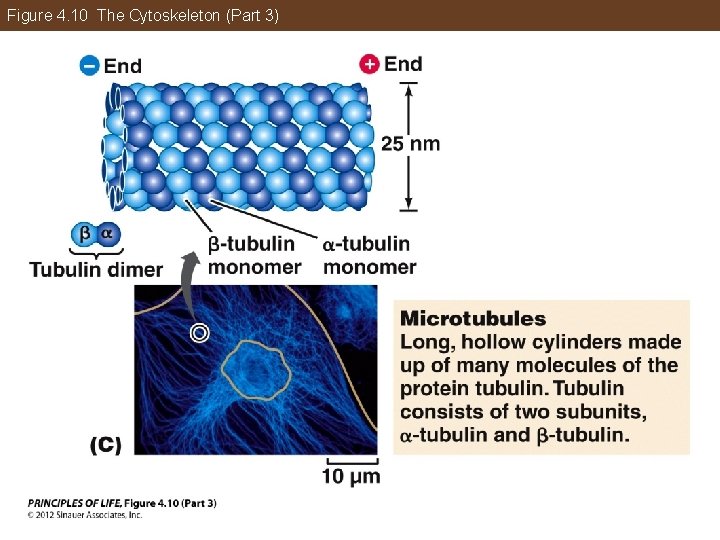
Figure 4. 10 The Cytoskeleton (Part 3)
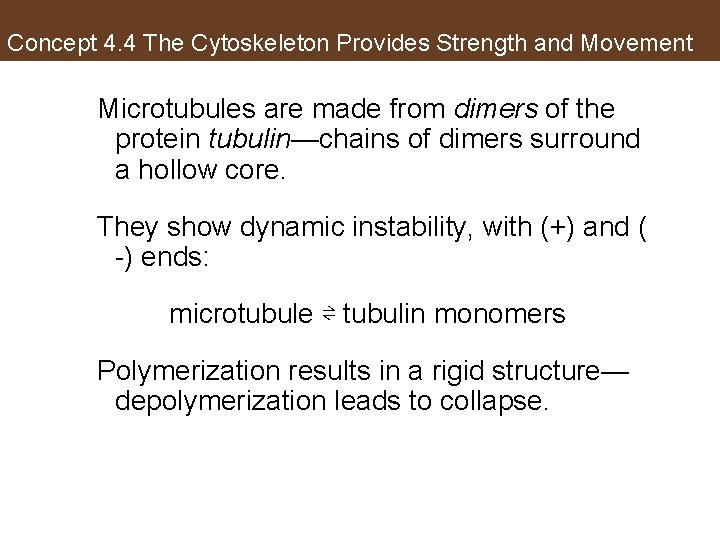
Concept 4. 4 The Cytoskeleton Provides Strength and Movement Microtubules are made from dimers of the protein tubulin—chains of dimers surround a hollow core. They show dynamic instability, with (+) and ( -) ends: microtubule ⇌ tubulin monomers Polymerization results in a rigid structure— depolymerization leads to collapse.
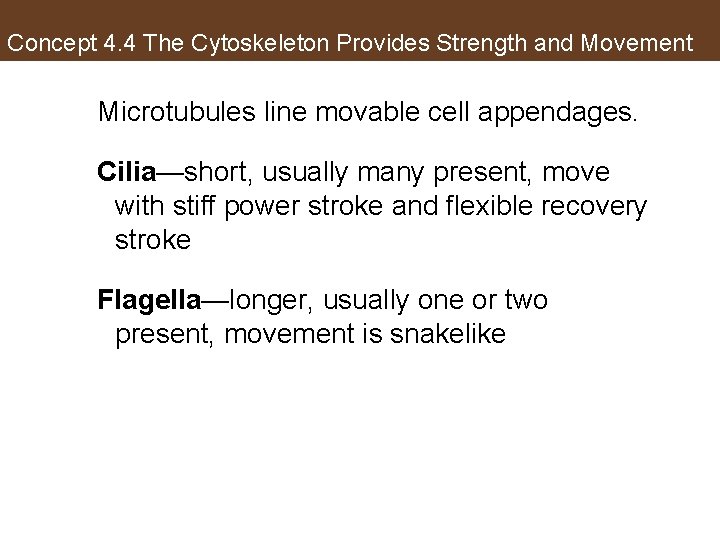
Concept 4. 4 The Cytoskeleton Provides Strength and Movement Microtubules line movable cell appendages. Cilia—short, usually many present, move with stiff power stroke and flexible recovery stroke Flagella—longer, usually one or two present, movement is snakelike
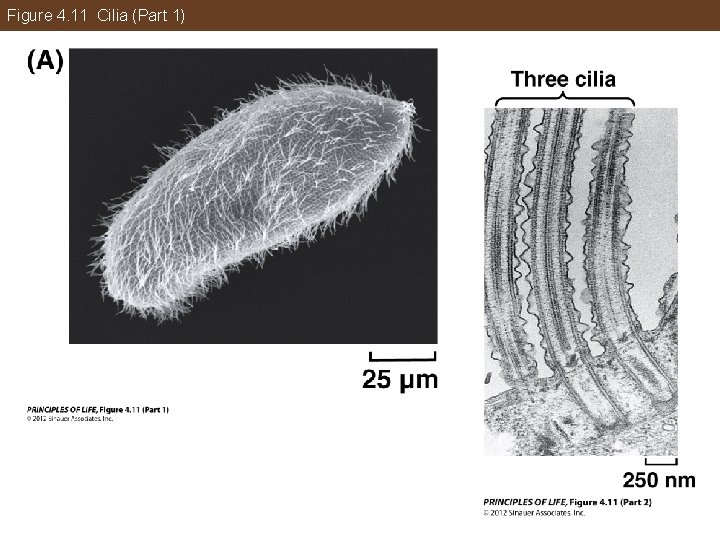
Figure 4. 11 Cilia (Part 1)
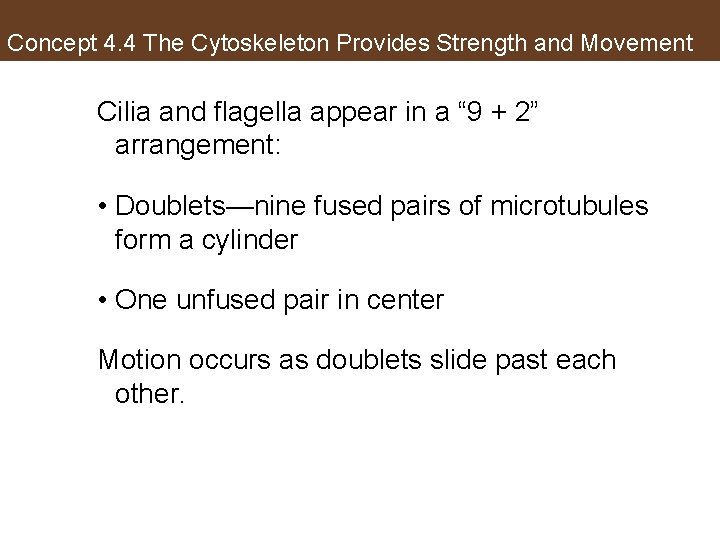
Concept 4. 4 The Cytoskeleton Provides Strength and Movement Cilia and flagella appear in a “ 9 + 2” arrangement: • Doublets—nine fused pairs of microtubules form a cylinder • One unfused pair in center Motion occurs as doublets slide past each other.
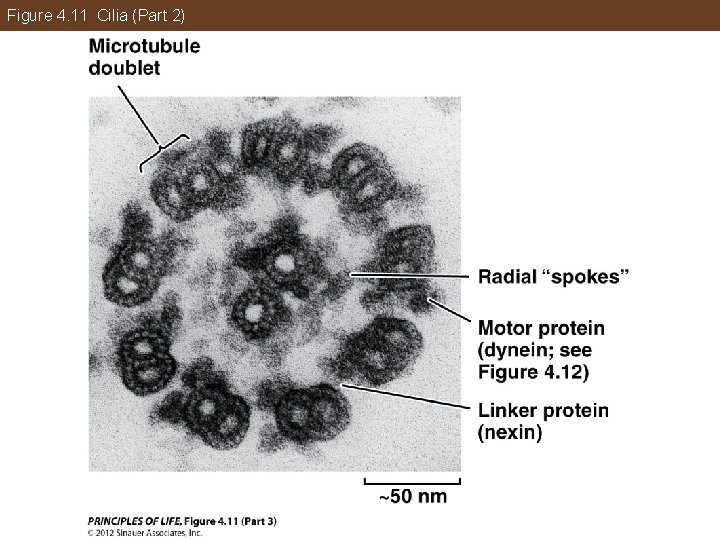
Figure 4. 11 Cilia (Part 2)
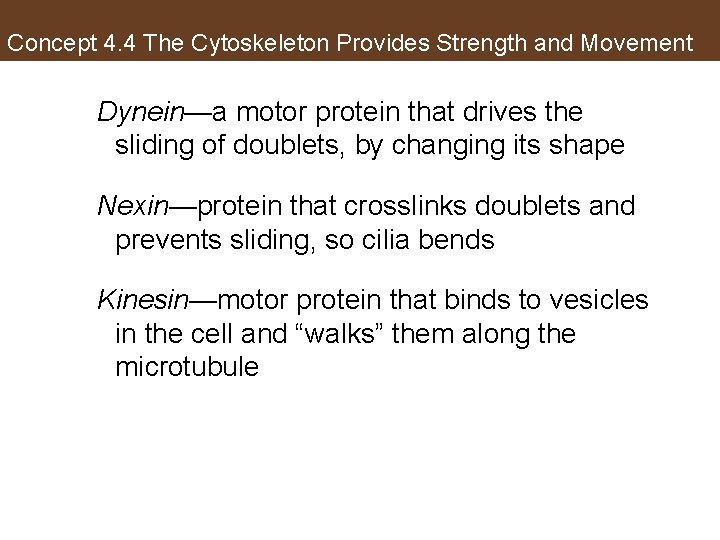
Concept 4. 4 The Cytoskeleton Provides Strength and Movement Dynein—a motor protein that drives the sliding of doublets, by changing its shape Nexin—protein that crosslinks doublets and prevents sliding, so cilia bends Kinesin—motor protein that binds to vesicles in the cell and “walks” them along the microtubule
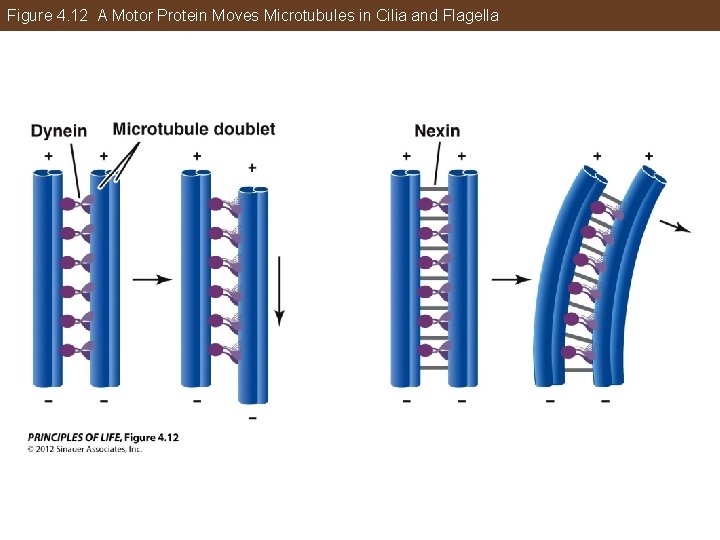
Figure 4. 12 A Motor Protein Moves Microtubules in Cilia and Flagella
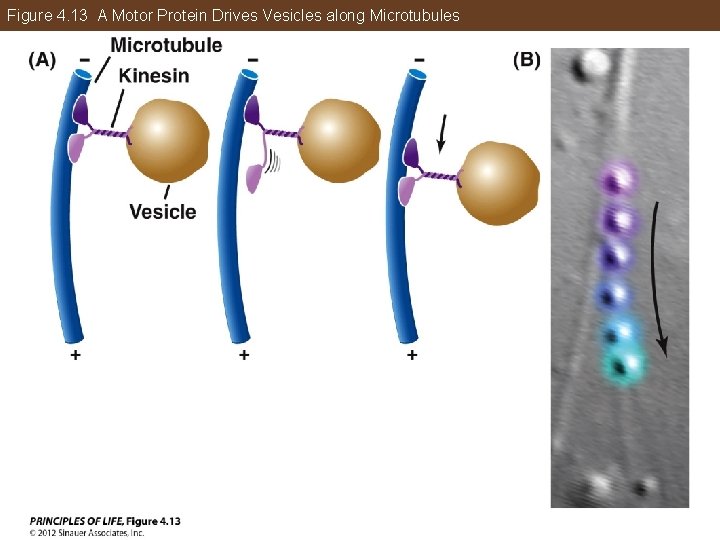
Figure 4. 13 A Motor Protein Drives Vesicles along Microtubules
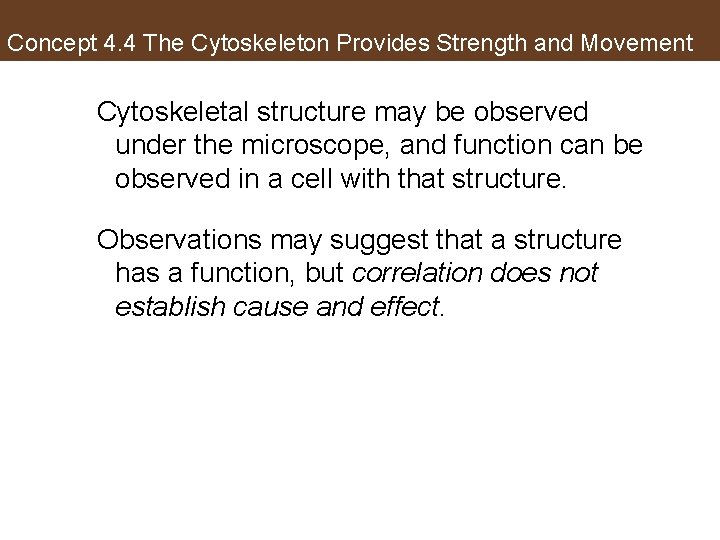
Concept 4. 4 The Cytoskeleton Provides Strength and Movement Cytoskeletal structure may be observed under the microscope, and function can be observed in a cell with that structure. Observations may suggest that a structure has a function, but correlation does not establish cause and effect.
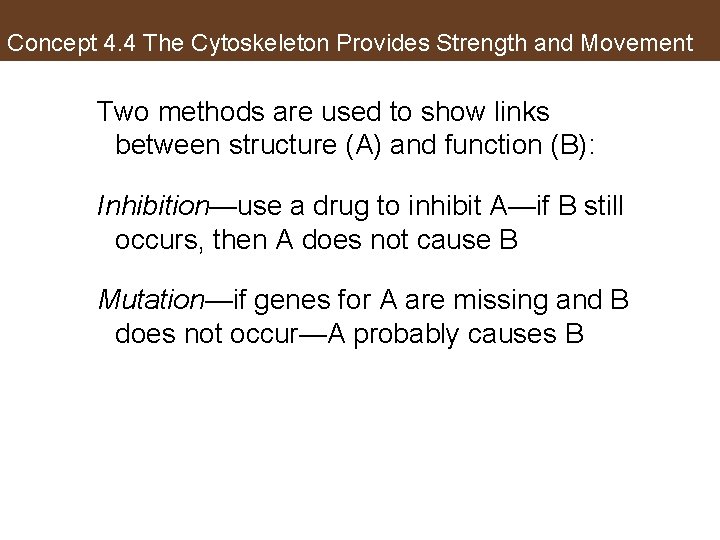
Concept 4. 4 The Cytoskeleton Provides Strength and Movement Two methods are used to show links between structure (A) and function (B): Inhibition—use a drug to inhibit A—if B still occurs, then A does not cause B Mutation—if genes for A are missing and B does not occur—A probably causes B
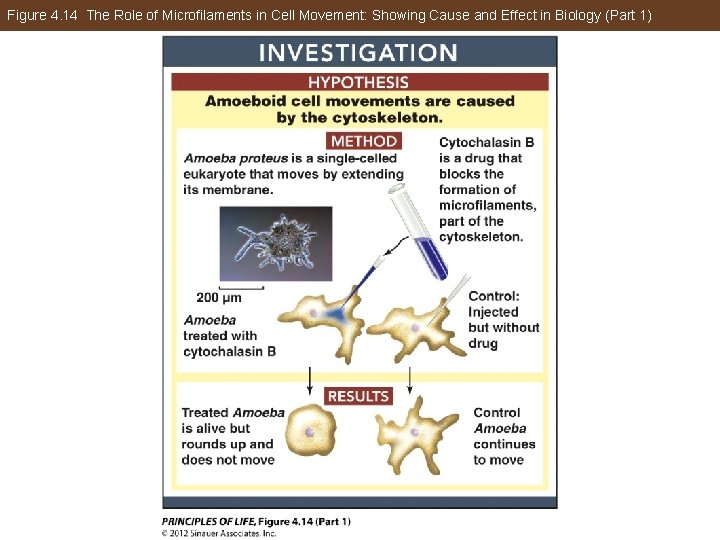
Figure 4. 14 The Role of Microfilaments in Cell Movement: Showing Cause and Effect in Biology (Part 1)
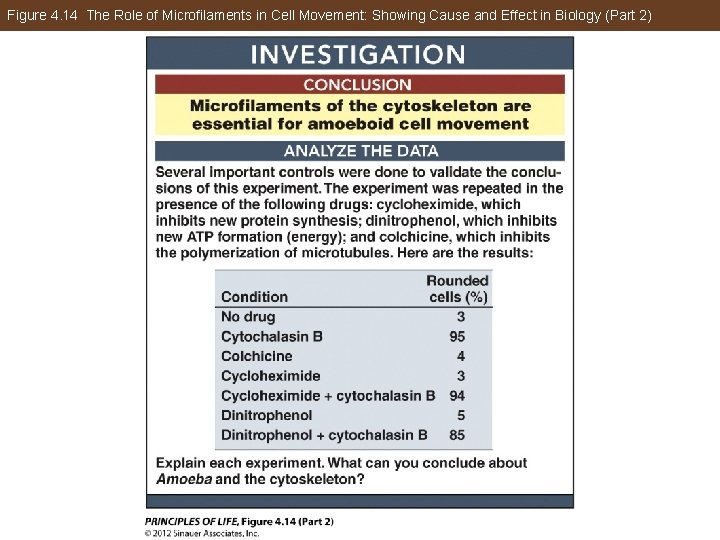
Figure 4. 14 The Role of Microfilaments in Cell Movement: Showing Cause and Effect in Biology (Part 2)
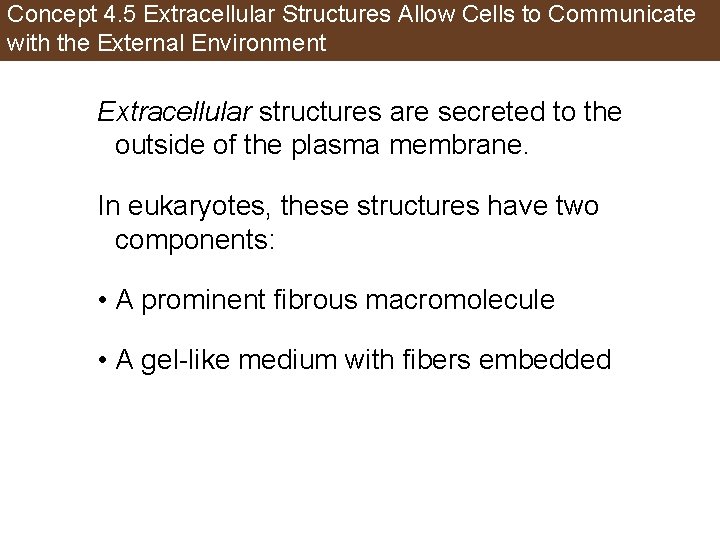
Concept 4. 5 Extracellular Structures Allow Cells to Communicate with the External Environment Extracellular structures are secreted to the outside of the plasma membrane. In eukaryotes, these structures have two components: • A prominent fibrous macromolecule • A gel-like medium with fibers embedded
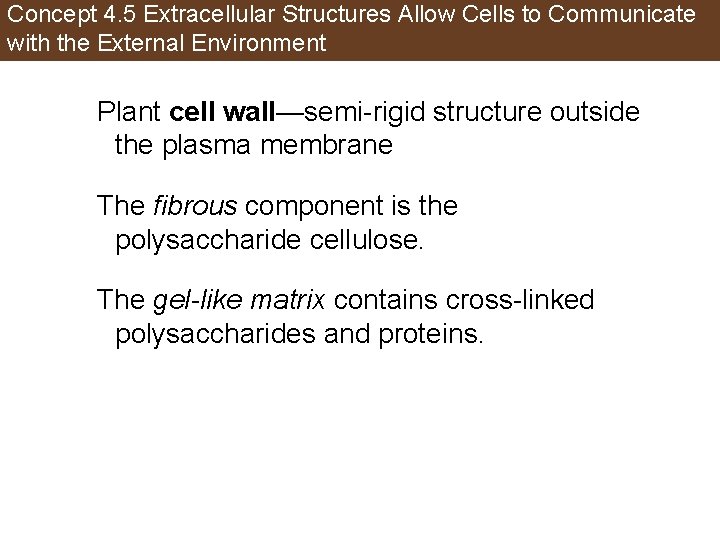
Concept 4. 5 Extracellular Structures Allow Cells to Communicate with the External Environment Plant cell wall—semi-rigid structure outside the plasma membrane The fibrous component is the polysaccharide cellulose. The gel-like matrix contains cross-linked polysaccharides and proteins.
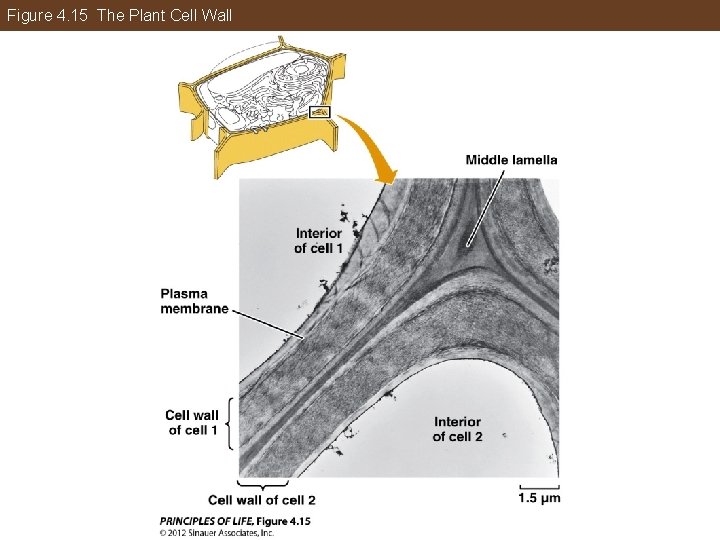
Figure 4. 15 The Plant Cell Wall
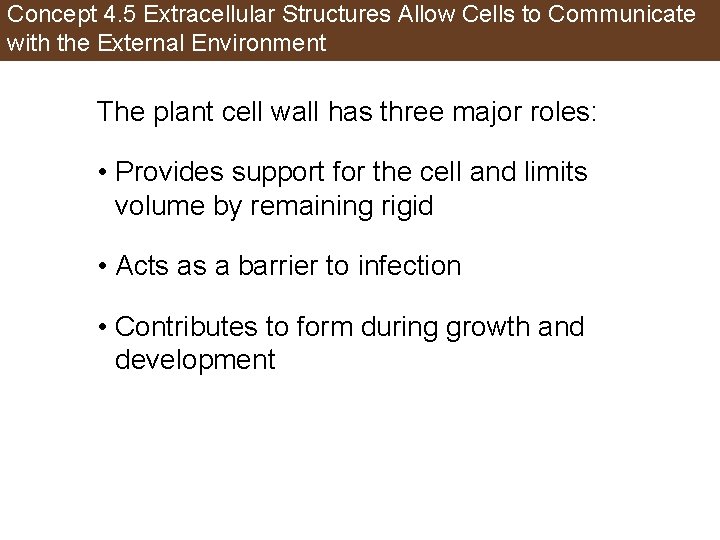
Concept 4. 5 Extracellular Structures Allow Cells to Communicate with the External Environment The plant cell wall has three major roles: • Provides support for the cell and limits volume by remaining rigid • Acts as a barrier to infection • Contributes to form during growth and development
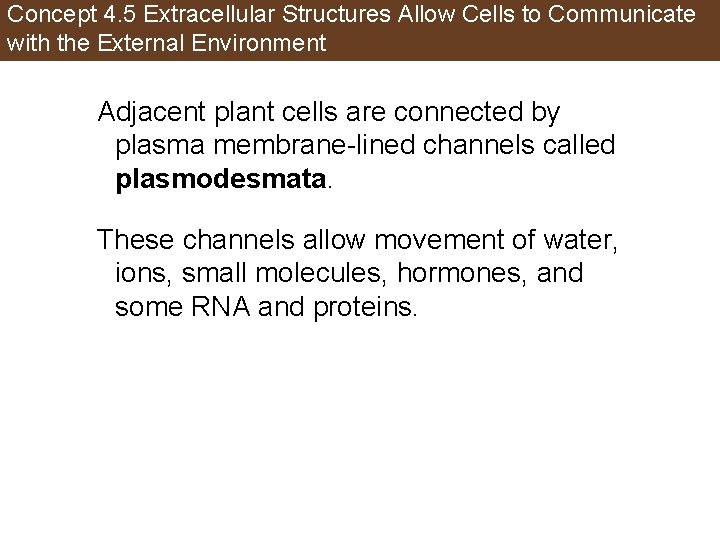
Concept 4. 5 Extracellular Structures Allow Cells to Communicate with the External Environment Adjacent plant cells are connected by plasma membrane-lined channels called plasmodesmata. These channels allow movement of water, ions, small molecules, hormones, and some RNA and proteins.
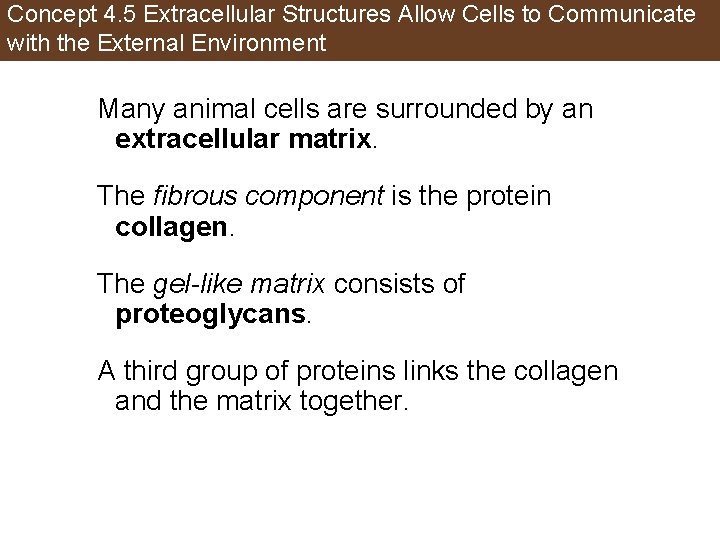
Concept 4. 5 Extracellular Structures Allow Cells to Communicate with the External Environment Many animal cells are surrounded by an extracellular matrix. The fibrous component is the protein collagen. The gel-like matrix consists of proteoglycans. A third group of proteins links the collagen and the matrix together.
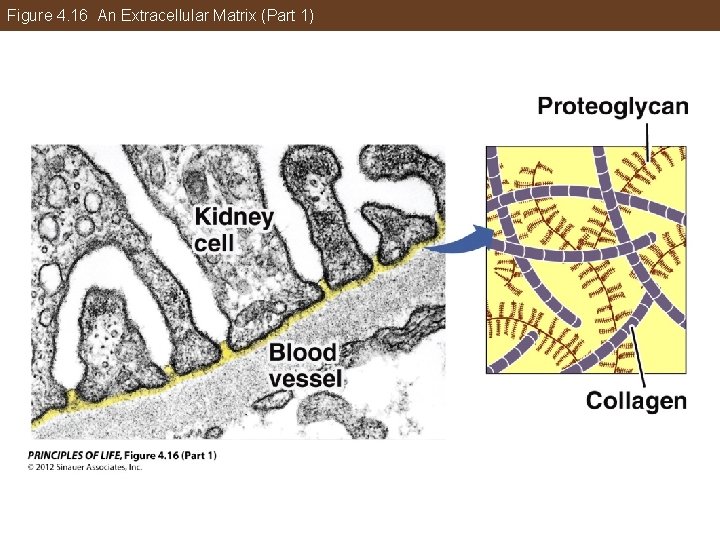
Figure 4. 16 An Extracellular Matrix (Part 1)
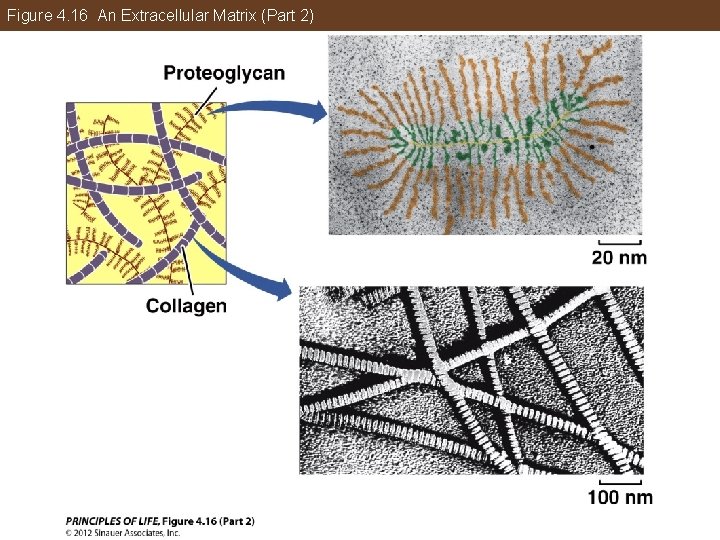
Figure 4. 16 An Extracellular Matrix (Part 2)
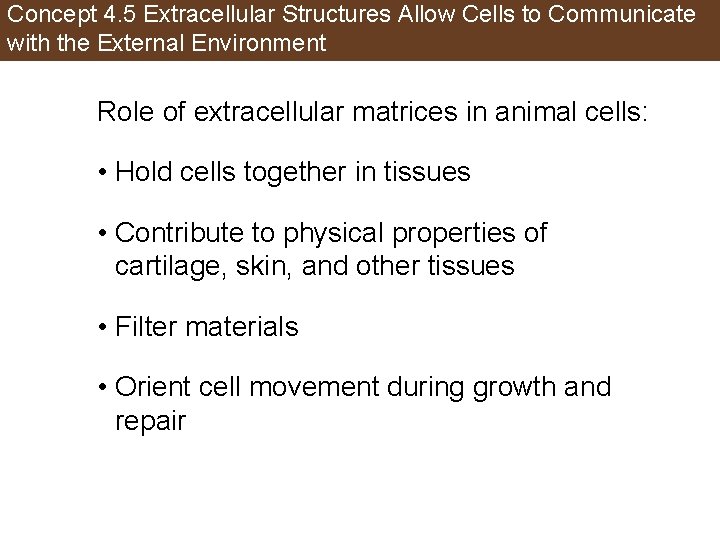
Concept 4. 5 Extracellular Structures Allow Cells to Communicate with the External Environment Role of extracellular matrices in animal cells: • Hold cells together in tissues • Contribute to physical properties of cartilage, skin, and other tissues • Filter materials • Orient cell movement during growth and repair
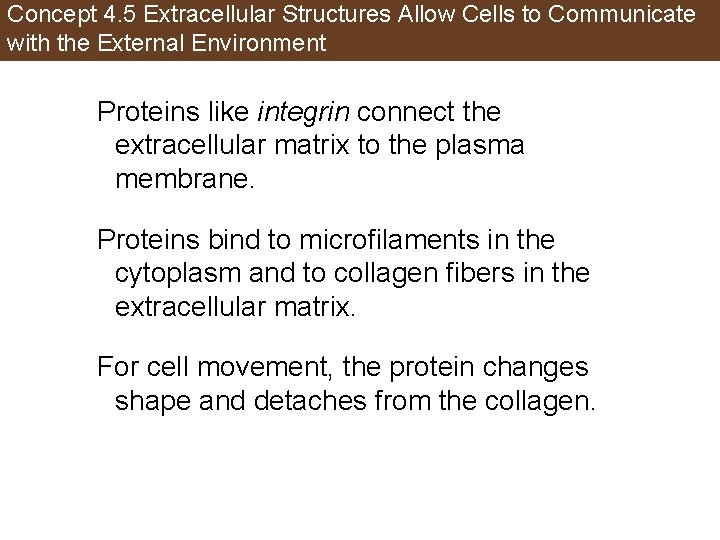
Concept 4. 5 Extracellular Structures Allow Cells to Communicate with the External Environment Proteins like integrin connect the extracellular matrix to the plasma membrane. Proteins bind to microfilaments in the cytoplasm and to collagen fibers in the extracellular matrix. For cell movement, the protein changes shape and detaches from the collagen.
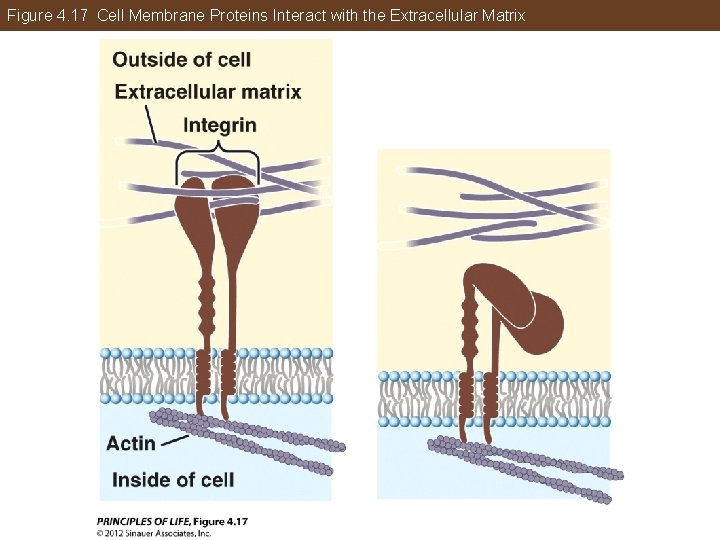
Figure 4. 17 Cell Membrane Proteins Interact with the Extracellular Matrix
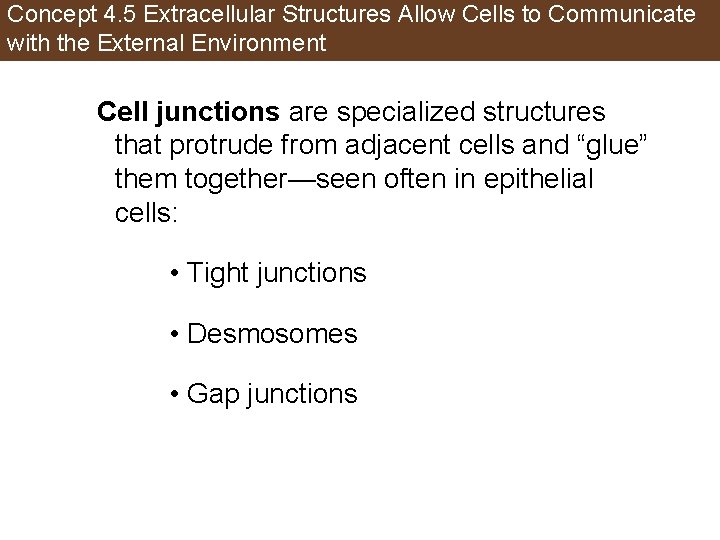
Concept 4. 5 Extracellular Structures Allow Cells to Communicate with the External Environment Cell junctions are specialized structures that protrude from adjacent cells and “glue” them together—seen often in epithelial cells: • Tight junctions • Desmosomes • Gap junctions
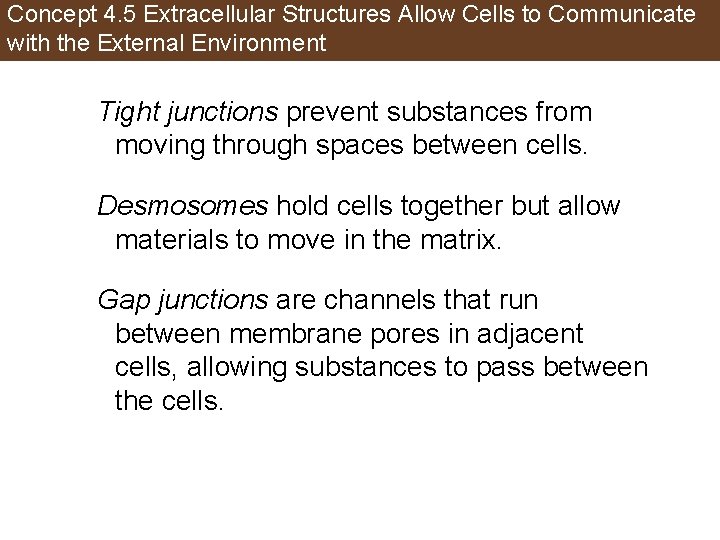
Concept 4. 5 Extracellular Structures Allow Cells to Communicate with the External Environment Tight junctions prevent substances from moving through spaces between cells. Desmosomes hold cells together but allow materials to move in the matrix. Gap junctions are channels that run between membrane pores in adjacent cells, allowing substances to pass between the cells.
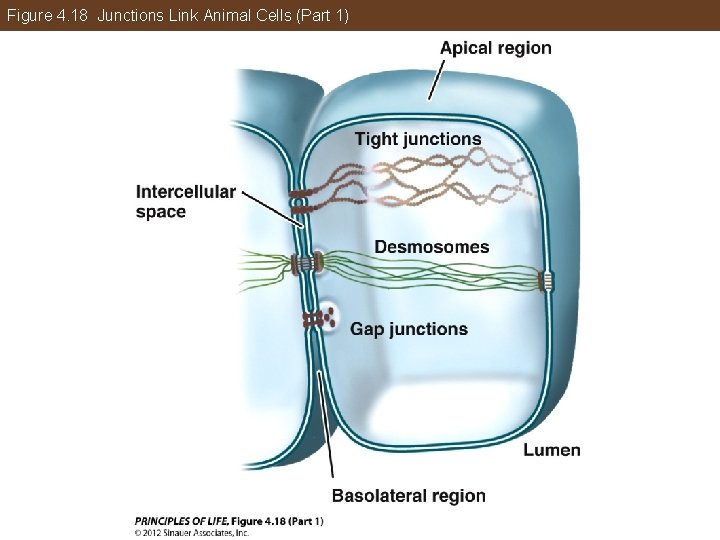
Figure 4. 18 Junctions Link Animal Cells (Part 1)
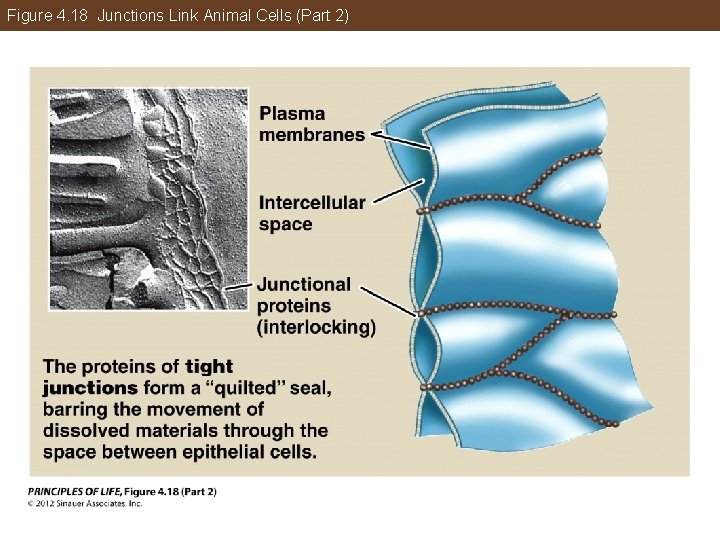
Figure 4. 18 Junctions Link Animal Cells (Part 2)
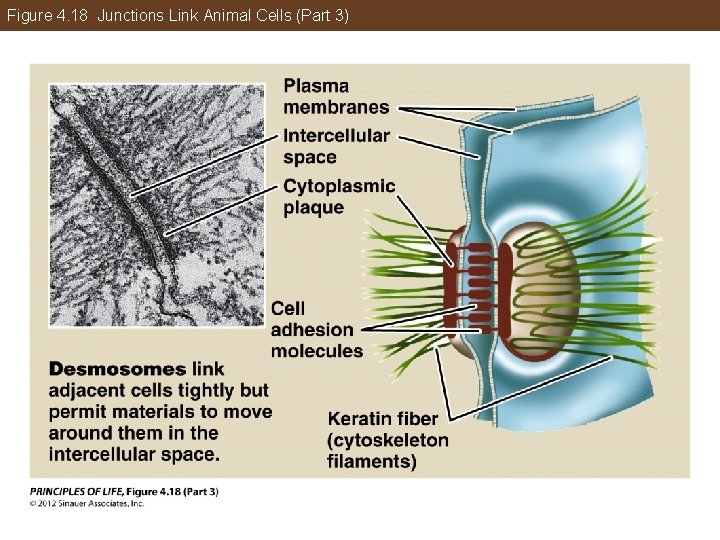
Figure 4. 18 Junctions Link Animal Cells (Part 3)
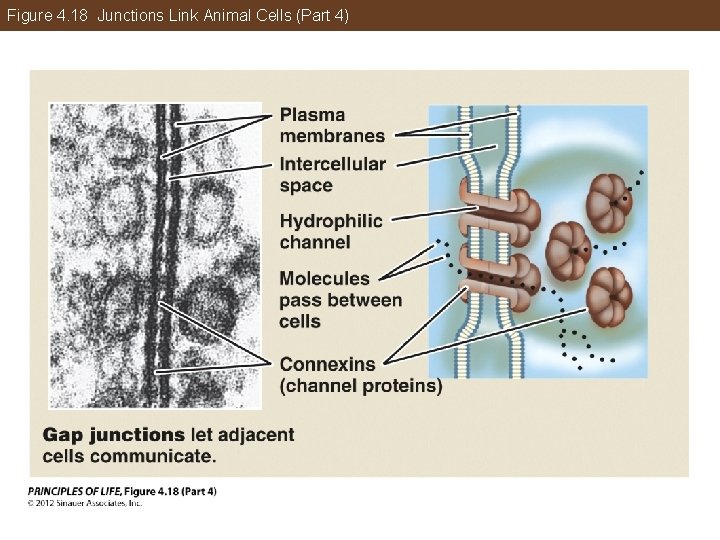
Figure 4. 18 Junctions Link Animal Cells (Part 4)
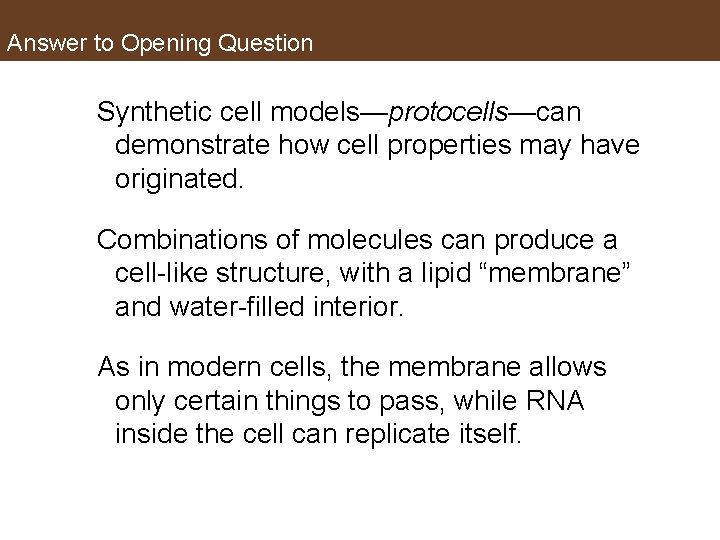
Answer to Opening Question Synthetic cell models—protocells—can demonstrate how cell properties may have originated. Combinations of molecules can produce a cell-like structure, with a lipid “membrane” and water-filled interior. As in modern cells, the membrane allows only certain things to pass, while RNA inside the cell can replicate itself.
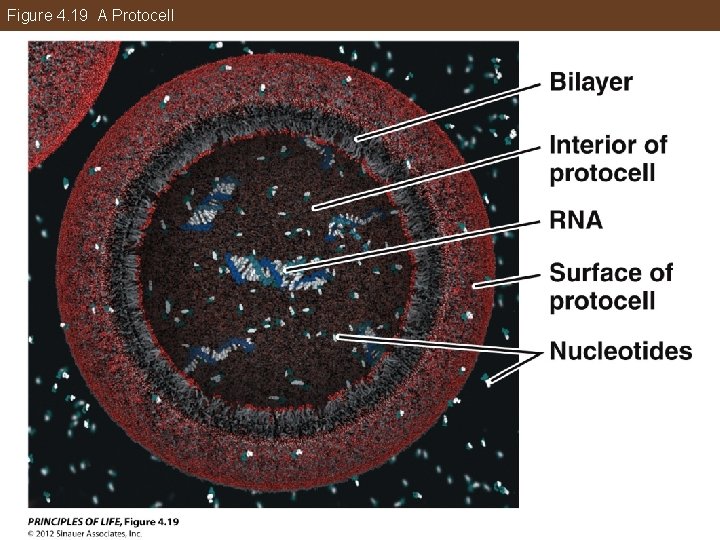
Figure 4. 19 A Protocell
Chapter 8 cellular reproduction cells from cells
Chapter 3 cells the living units
Chapter 3 cells the living units
Mitosis
Olfactory groove keros classification
Tubular lumen
Parafollicular
Somatic vs gamete
Why dna is more stable than rna
Chlorocruorin
Eukaryota
Venn diagram plant vs animal cells
Prokaryotes vs eukaryotes venn diagram
Organelle trail
Masses of cells form and steal nutrients from healthy cells
Pseudostratified vs simple columnar
What cell type
Is a red blood cell prokaryotic or eukaryotic
Cells cells they're made of organelles meme
A group of similar cells working together.
Tissues are groups of similar cells working together to
Tissues are groups of similar cells working together to
Group of cells working together
A group of cells similar in structure and function
Hard work vs smart work presentation
Hot working
Hot working and cold working difference
Differentiate between hot working and cold working
Proses pengerjaan panas
Speywood units vs botox units
Absorption costing income statement
The smallest unit of life is the
Cells are the smallest unit of life
Cells and life lesson 1 answer key
A cell is the smallest unit of
True or false questions about cells
The life of a hard-working future king
Working capital life cycle
Work studies core my working life answers
Importance of physics
The basic units of life
Hát kết hợp bộ gõ cơ thể
Lp html
Bổ thể
Tỉ lệ cơ thể trẻ em
Gấu đi như thế nào
Glasgow thang điểm
Chúa yêu trần thế alleluia
Các môn thể thao bắt đầu bằng tiếng nhảy
Thế nào là hệ số cao nhất
Các châu lục và đại dương trên thế giới
Công thức tính thế năng
Trời xanh đây là của chúng ta thể thơ
Mật thư tọa độ 5x5
Làm thế nào để 102-1=99
độ dài liên kết
Các châu lục và đại dương trên thế giới
Thể thơ truyền thống
Quá trình desamine hóa có thể tạo ra
Một số thể thơ truyền thống
Cái miệng xinh xinh thế chỉ nói điều hay thôi
Vẽ hình chiếu vuông góc của vật thể sau
Thế nào là sự mỏi cơ
đặc điểm cơ thể của người tối cổ
Giọng cùng tên là
Vẽ hình chiếu đứng bằng cạnh của vật thể
Fecboak
Thẻ vin
đại từ thay thế
điện thế nghỉ
Tư thế ngồi viết
Diễn thế sinh thái là
Các loại đột biến cấu trúc nhiễm sắc thể
Các số nguyên tố
Tư thế ngồi viết
Lời thề hippocrates
Thiếu nhi thế giới liên hoan
ưu thế lai là gì
Hổ đẻ mỗi lứa mấy con
Sự nuôi và dạy con của hổ
Hệ hô hấp
Từ ngữ thể hiện lòng nhân hậu
Thế nào là mạng điện lắp đặt kiểu nổi
Chapter 6 how cells harvest chemical energy
Body tissue
Body tissues chapter 3 cells and tissues
Chapter 3 cells and tissues figure 3-7
Cell membrane phospholipids
Body tissues chapter 3 cells and tissues
Smooth endoplasmic
Body tissues chapter 3 cells and tissues
Chapter 4 cells and energy
Chapter 6 how cells harvest chemical energy