Superconducting electronics from Josephson effects to quantum computing
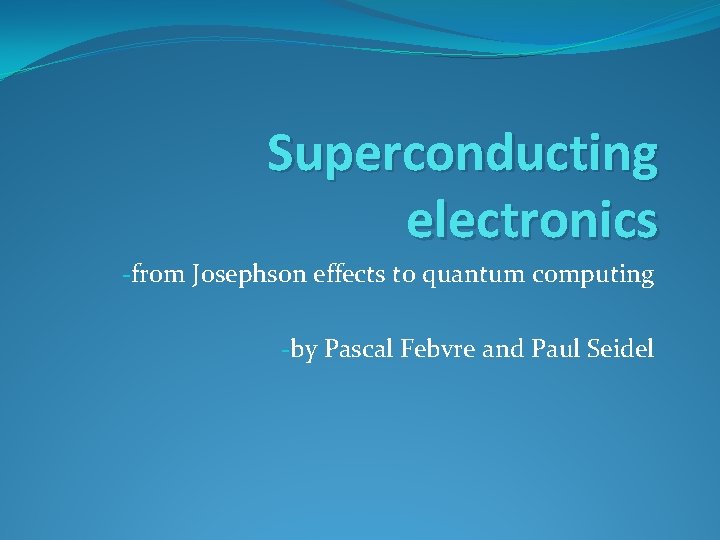
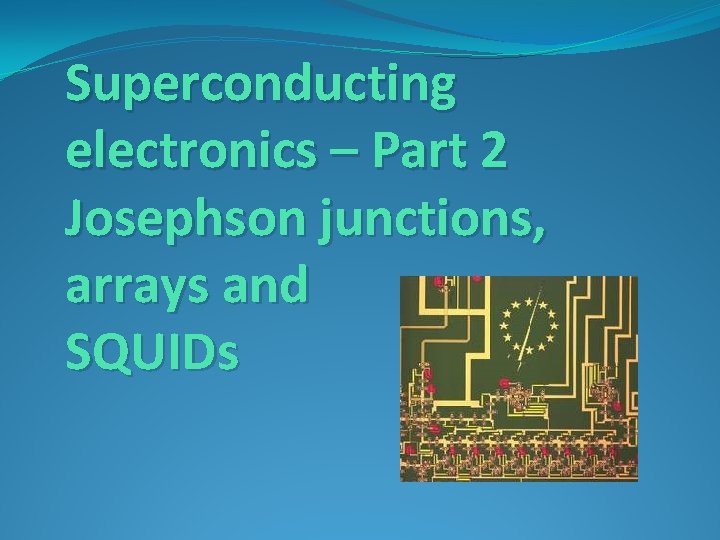
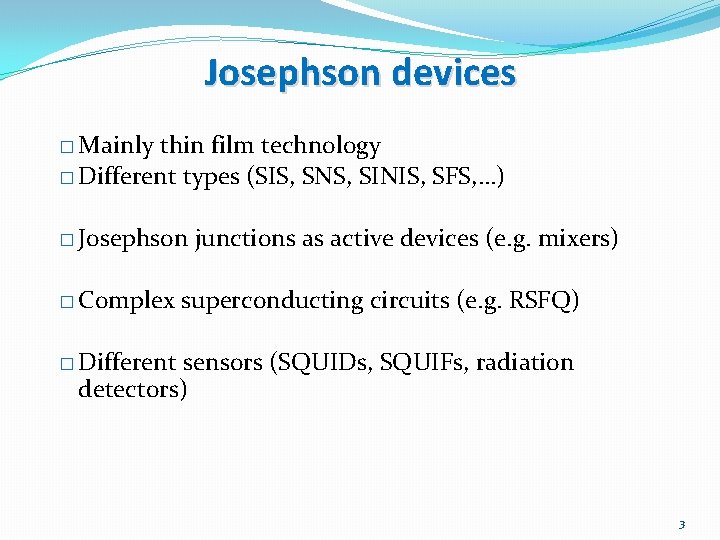
![Thin film preparation methods [R. Wördenweber] Thin film preparation methods [R. Wördenweber]](https://slidetodoc.com/presentation_image_h2/81def57c997796372a709fb5fc171f16/image-4.jpg)
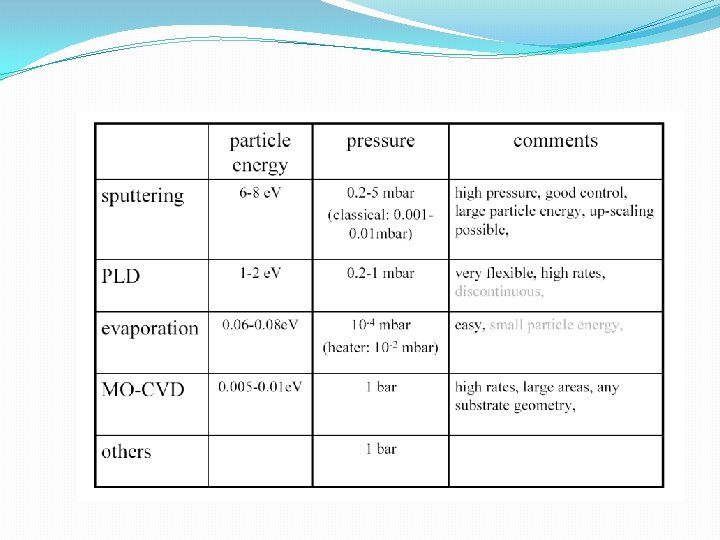
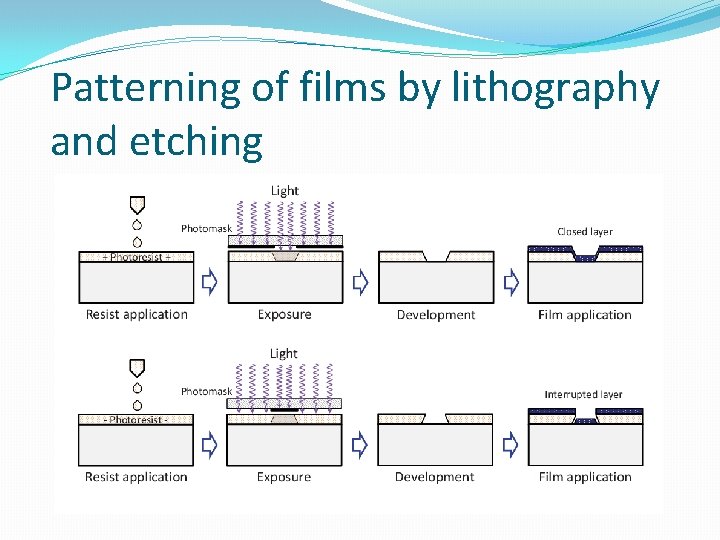
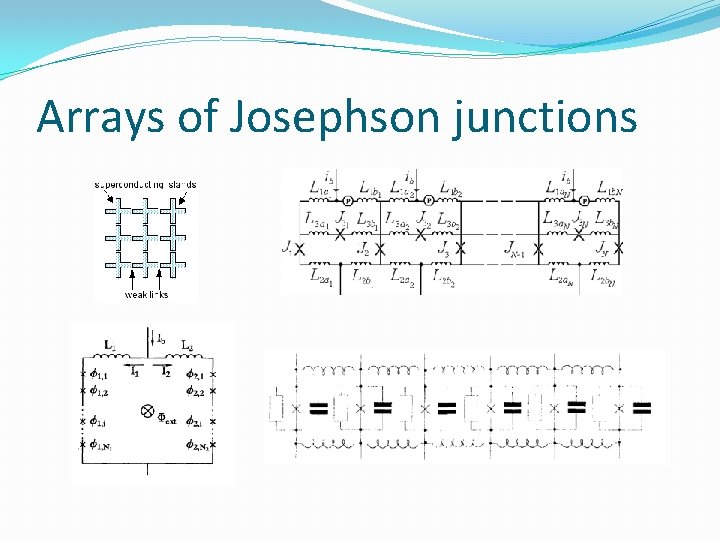
![Serial arrays for voltage standard [wikipedia] Serial arrays for voltage standard [wikipedia]](https://slidetodoc.com/presentation_image_h2/81def57c997796372a709fb5fc171f16/image-8.jpg)
![[ J. Niemeier ] 9 [ J. Niemeier ] 9](https://slidetodoc.com/presentation_image_h2/81def57c997796372a709fb5fc171f16/image-9.jpg)
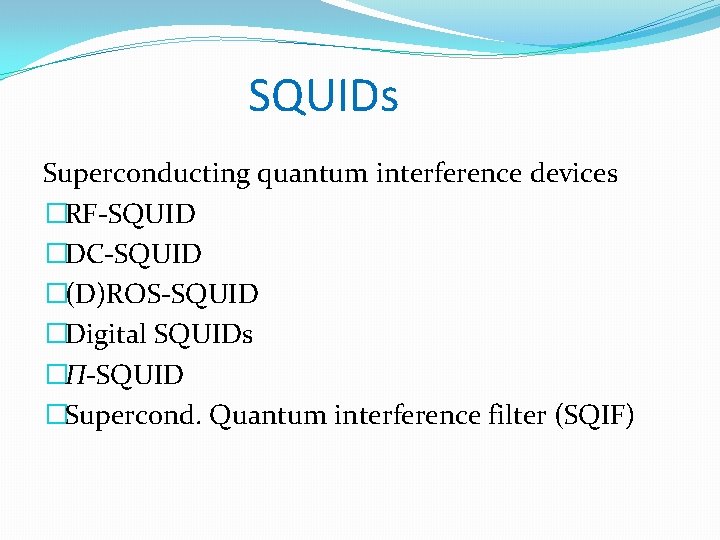
![[Gallop] [Gallop]](https://slidetodoc.com/presentation_image_h2/81def57c997796372a709fb5fc171f16/image-11.jpg)
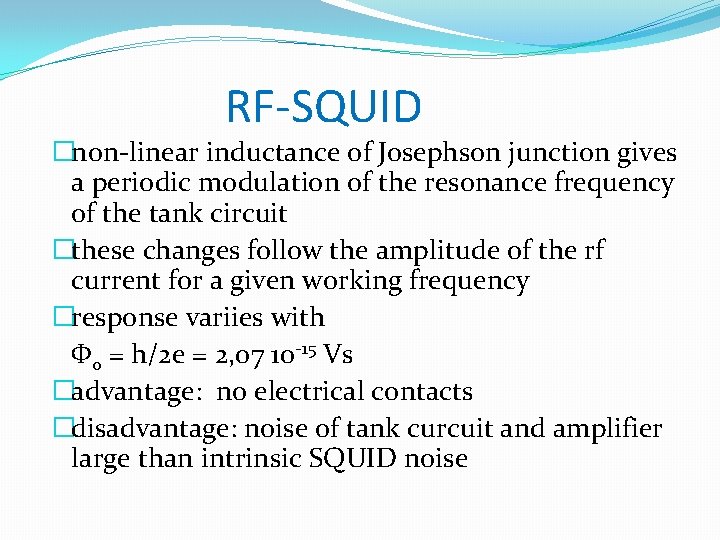
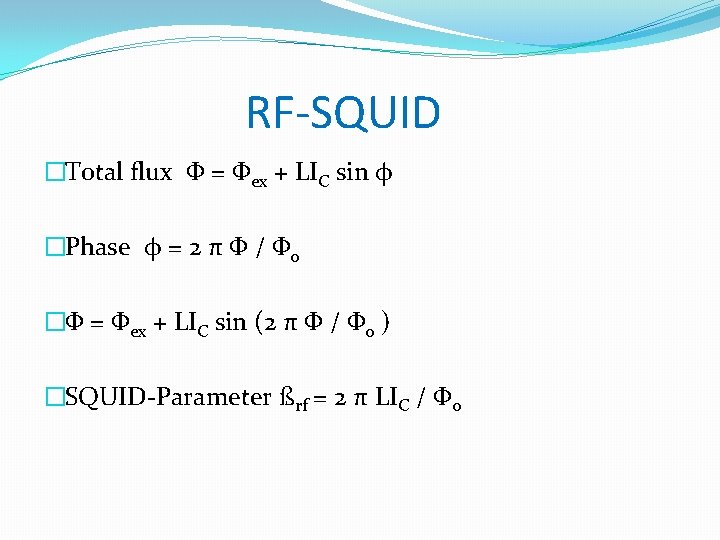
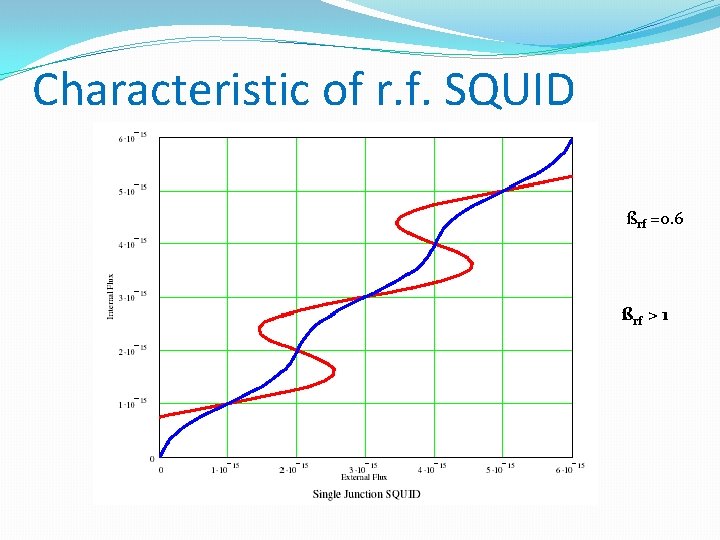
![LIC = 1. 25 Φ 0 k = 0 ↔ k =1 [Ruggiero, Rudman] LIC = 1. 25 Φ 0 k = 0 ↔ k =1 [Ruggiero, Rudman]](https://slidetodoc.com/presentation_image_h2/81def57c997796372a709fb5fc171f16/image-15.jpg)
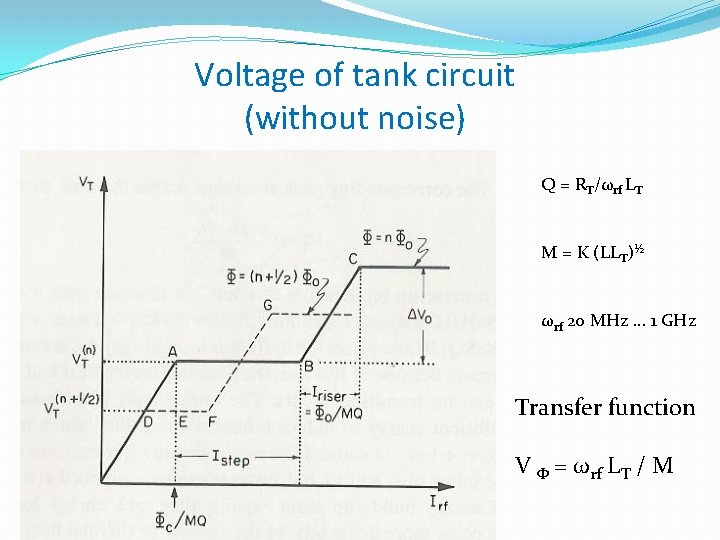
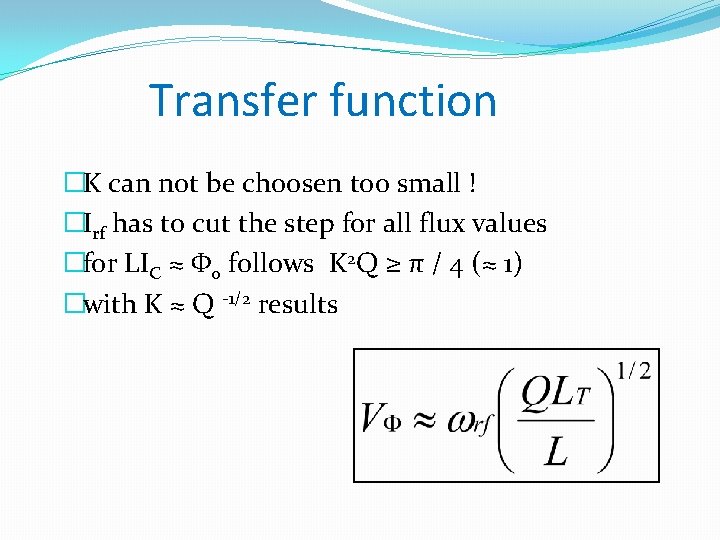
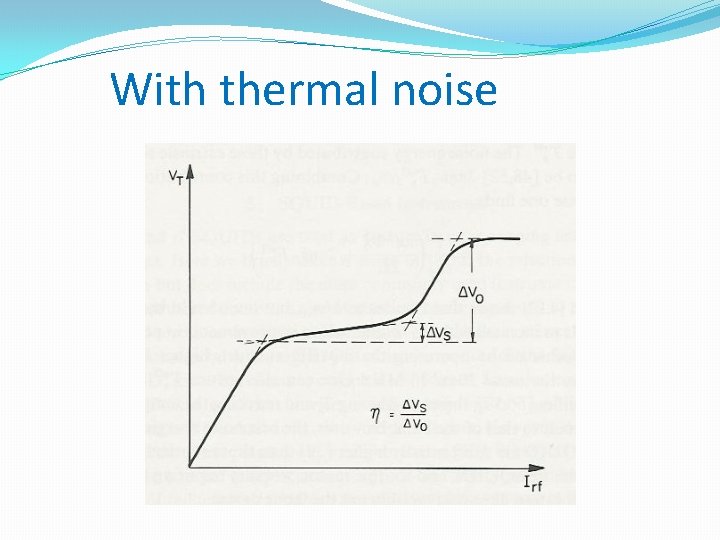
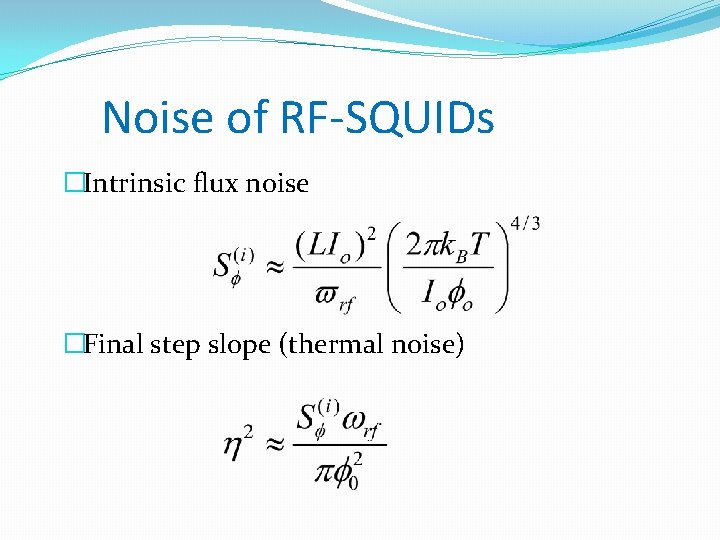
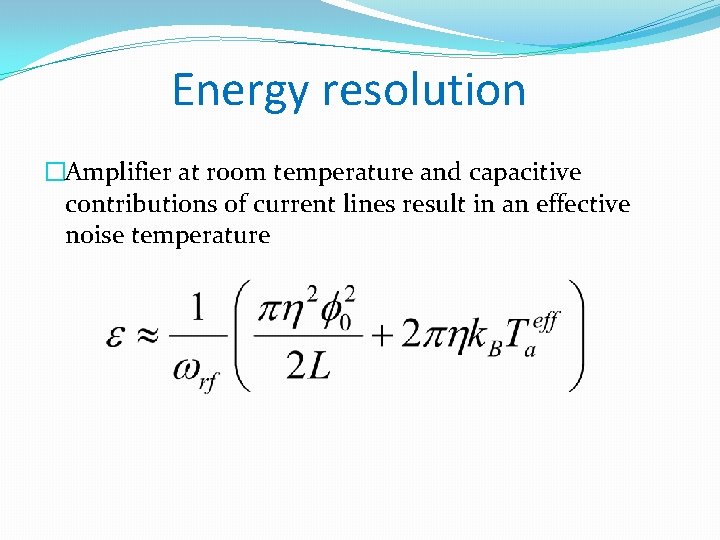
![Parameter [SQUID Handbook] Parameter [SQUID Handbook]](https://slidetodoc.com/presentation_image_h2/81def57c997796372a709fb5fc171f16/image-21.jpg)
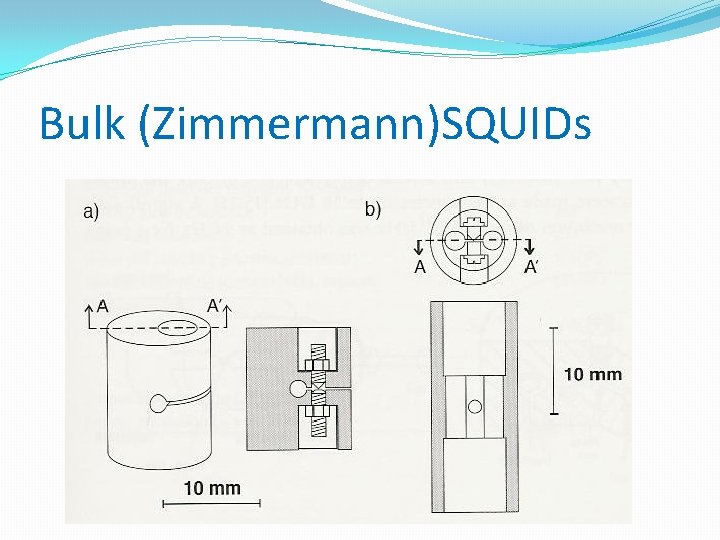
![Transition to films [SQUID Handbook] Transition to films [SQUID Handbook]](https://slidetodoc.com/presentation_image_h2/81def57c997796372a709fb5fc171f16/image-23.jpg)
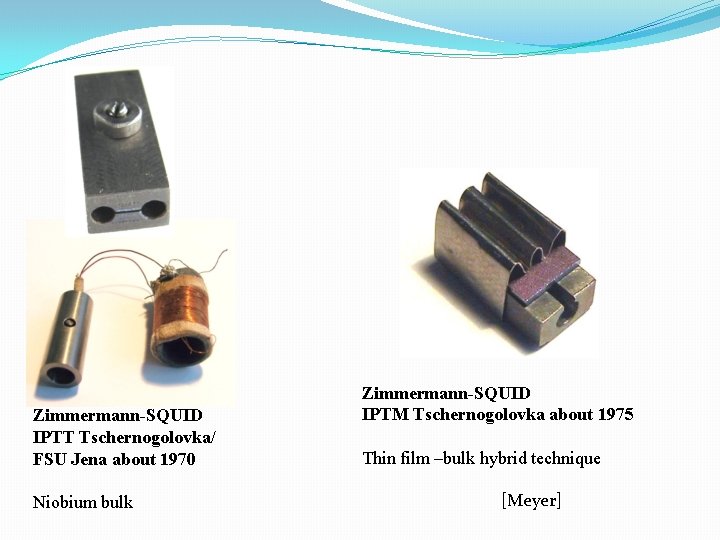
![HTS-RF-SQUID [FZ Jülich] HTS-RF-SQUID [FZ Jülich]](https://slidetodoc.com/presentation_image_h2/81def57c997796372a709fb5fc171f16/image-25.jpg)
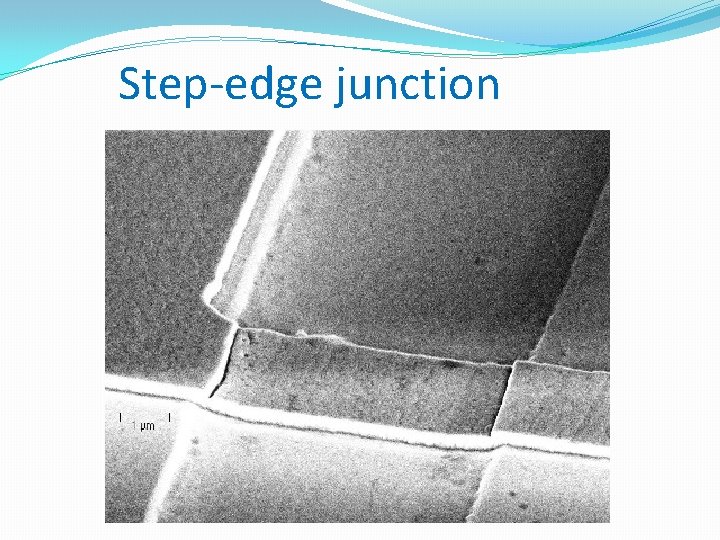
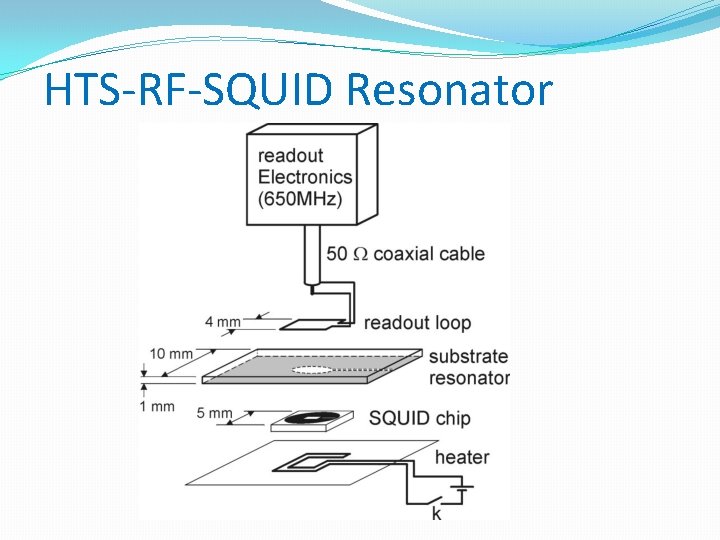
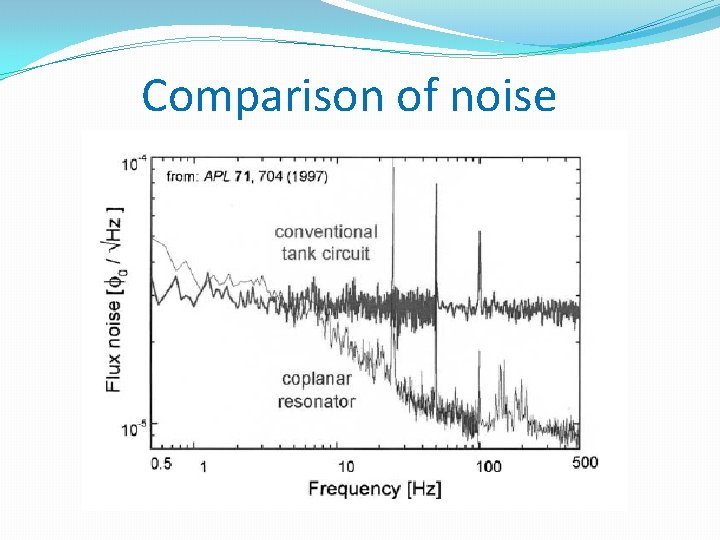
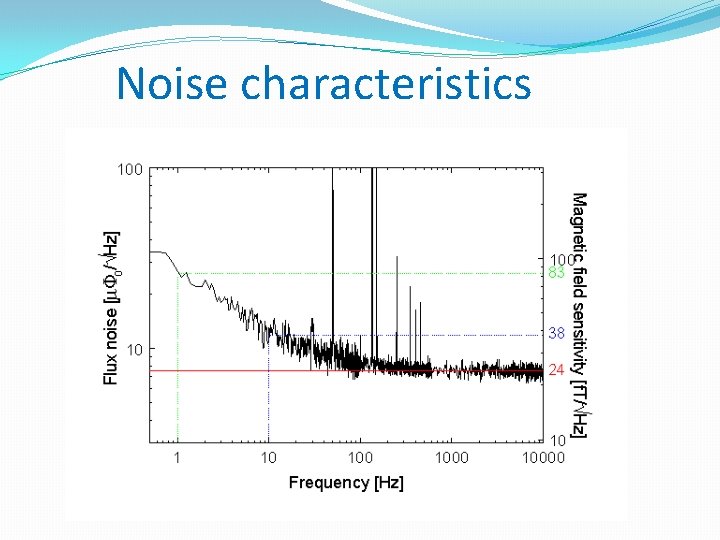
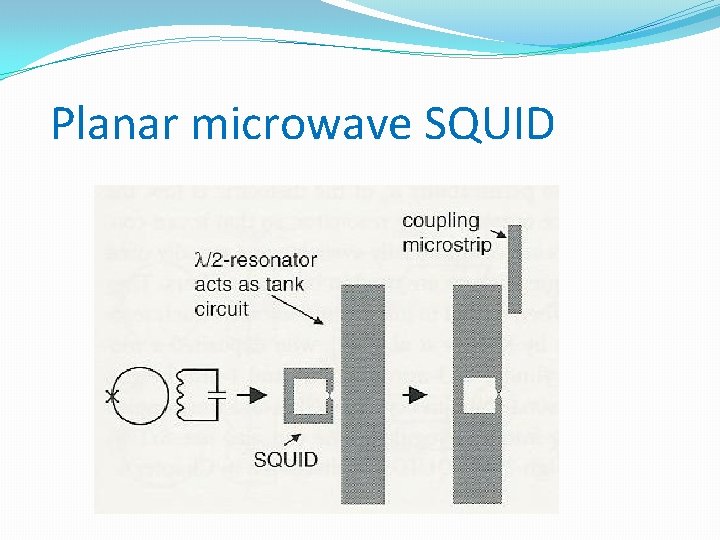
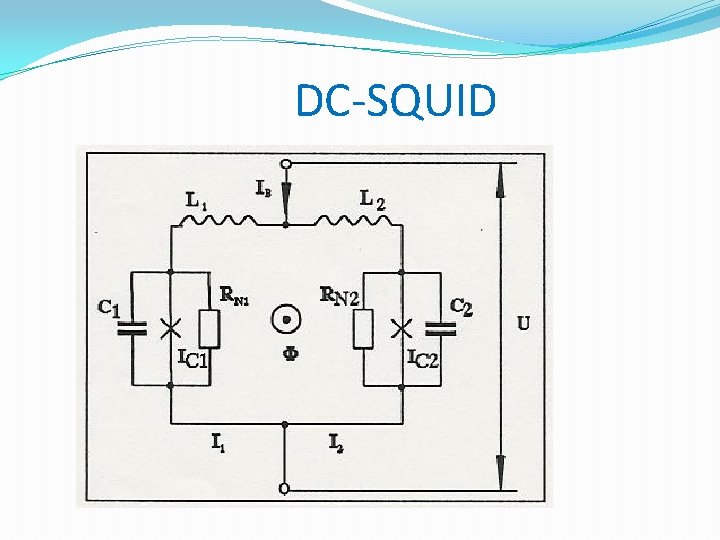
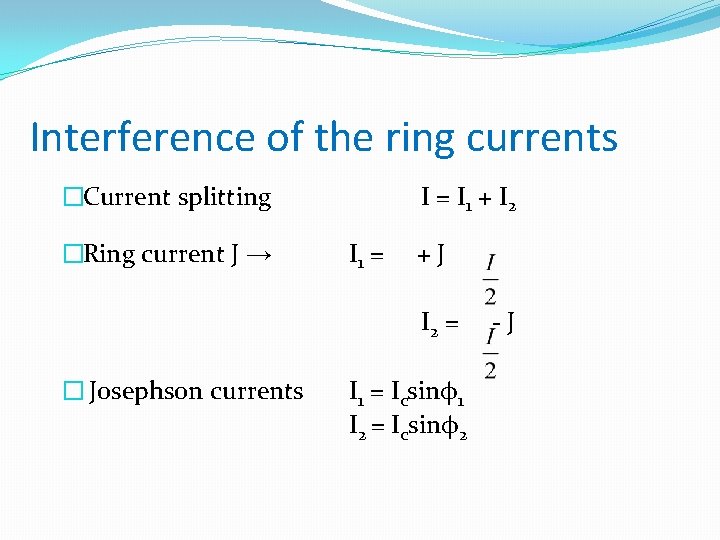
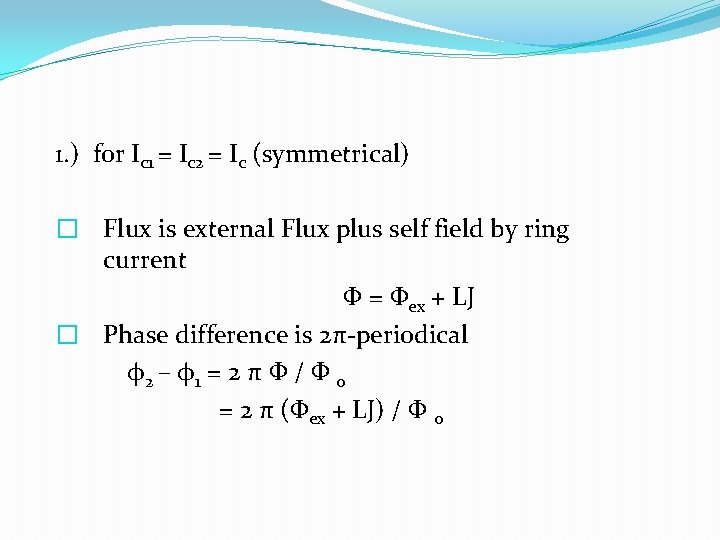
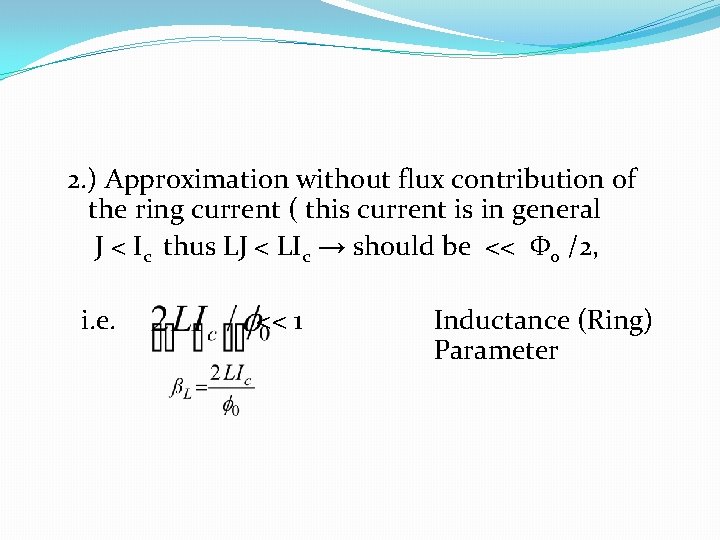
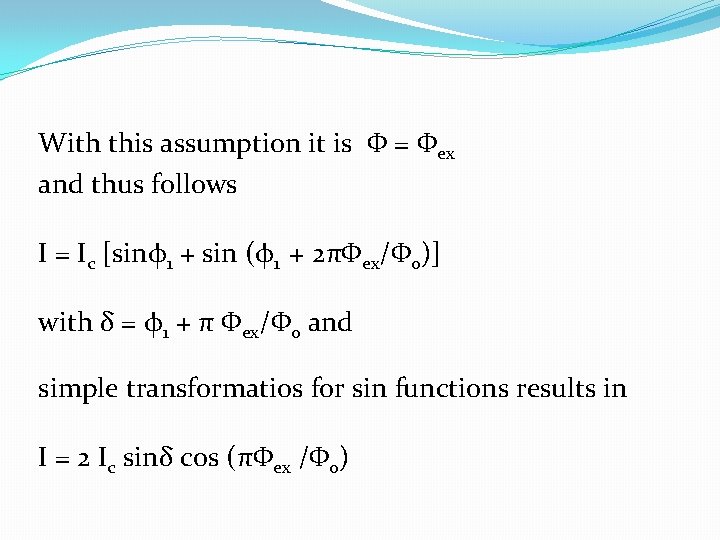
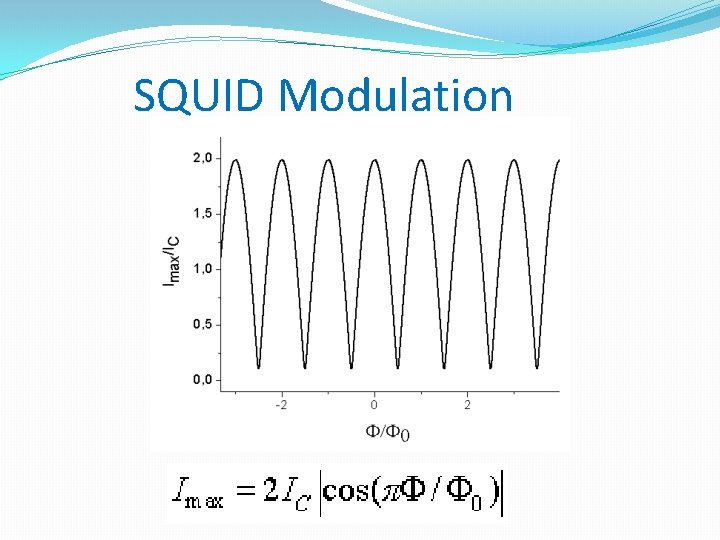
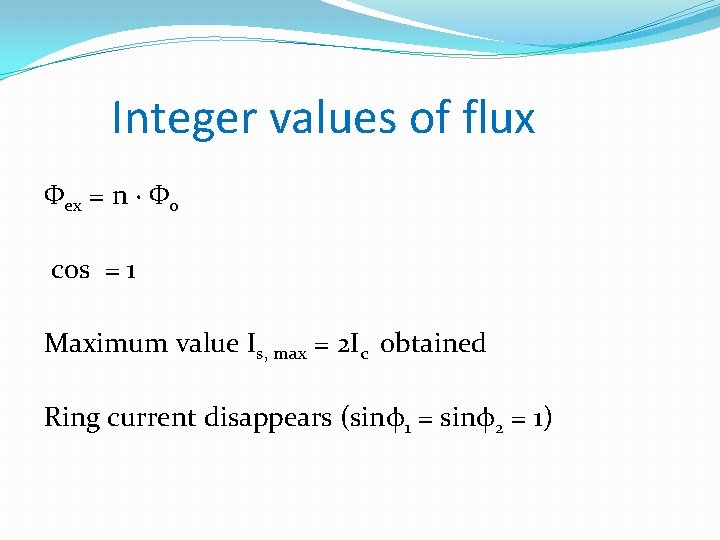
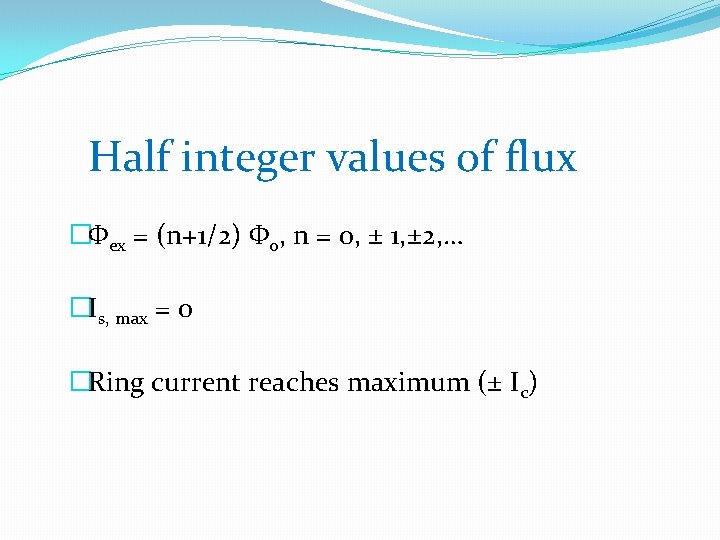
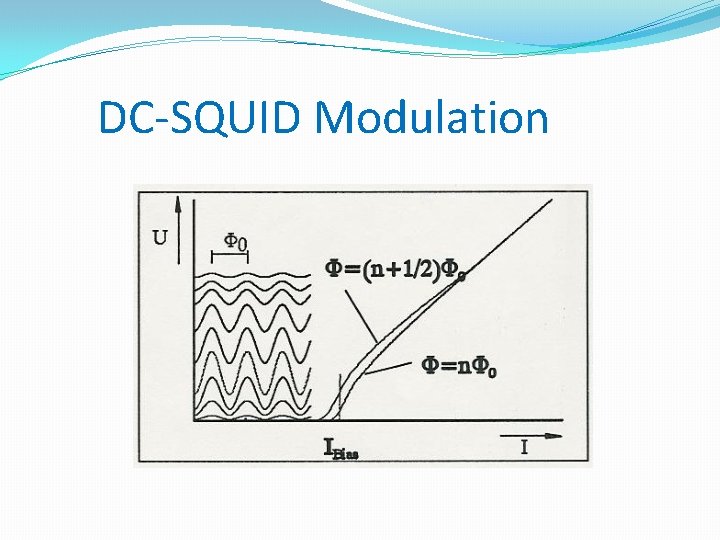
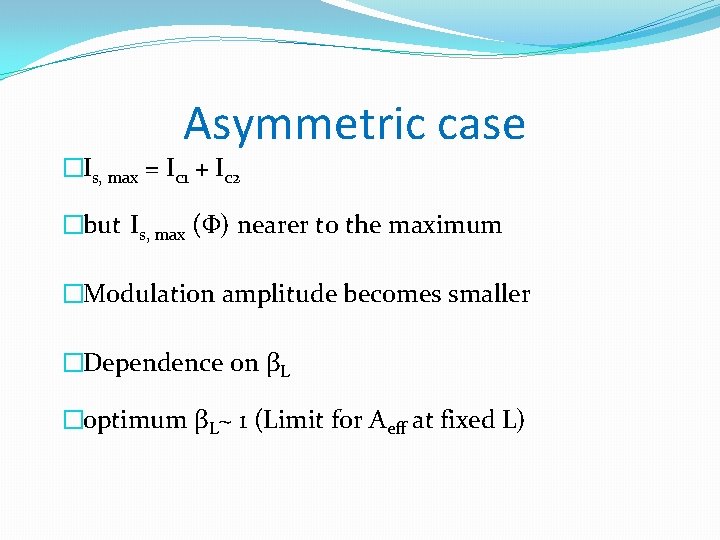
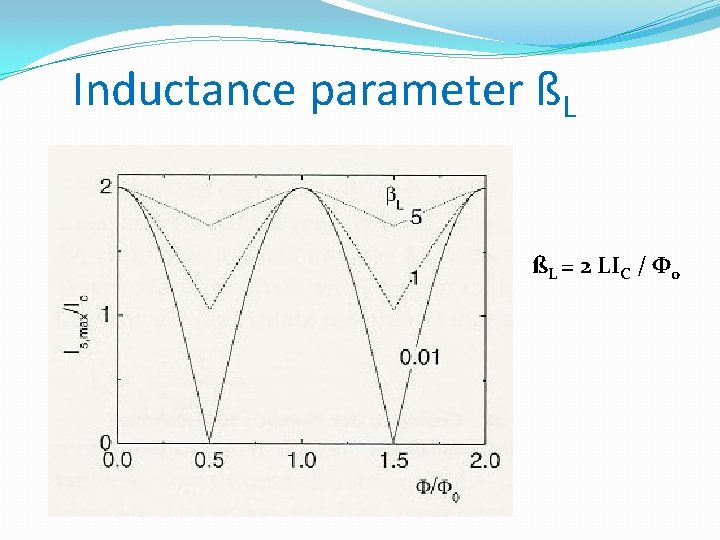
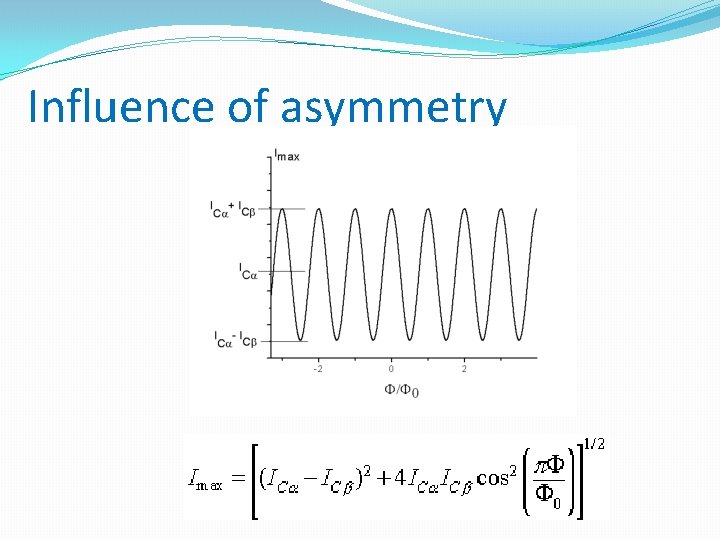
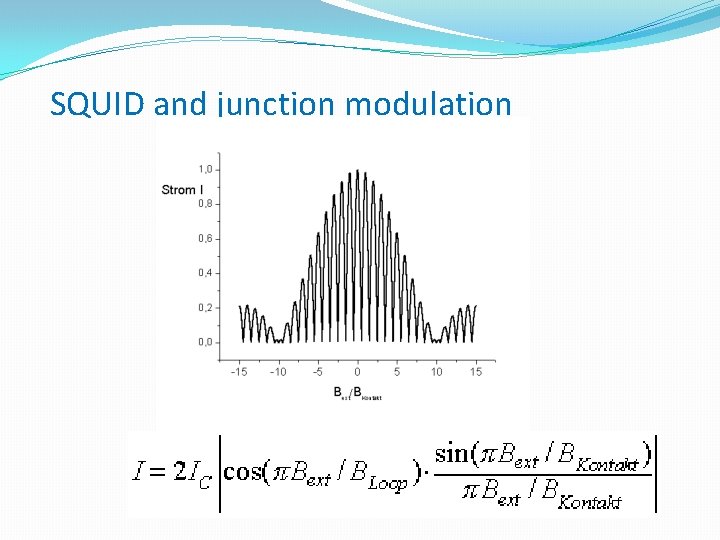
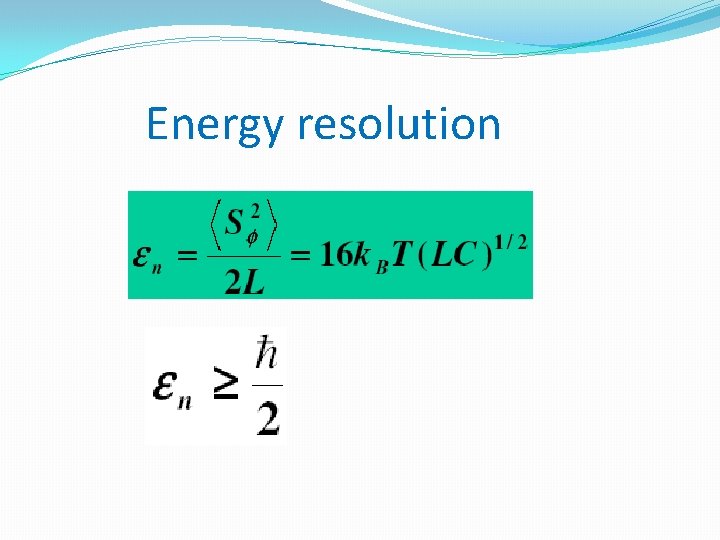
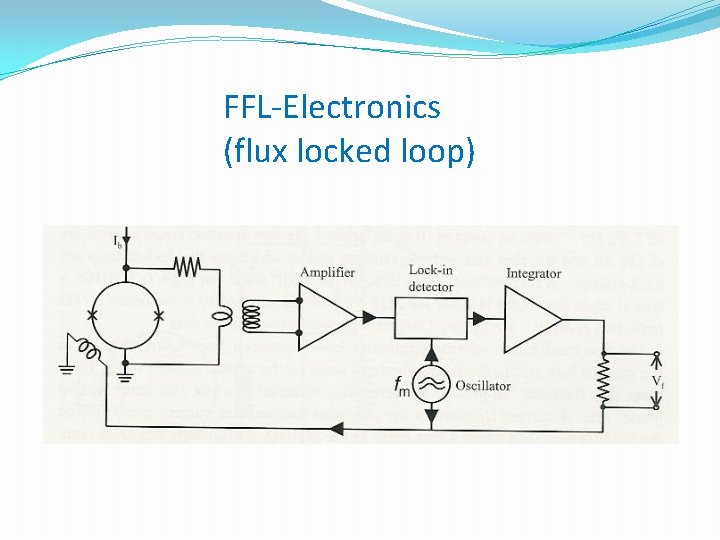
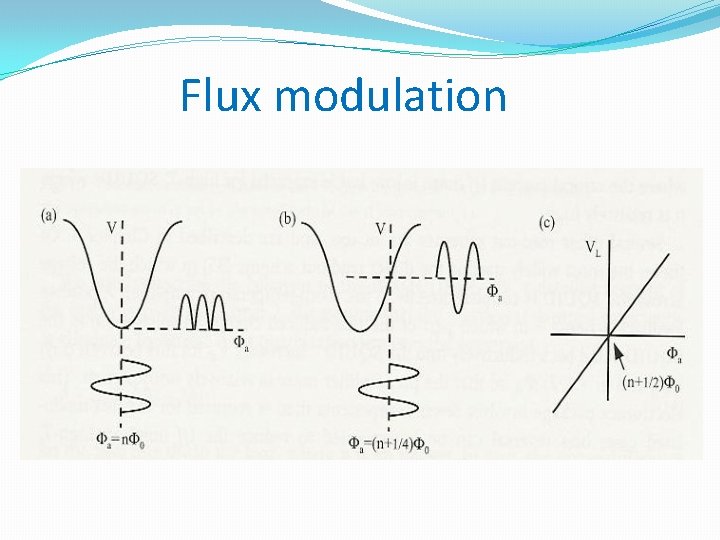
![[Drung] [Drung]](https://slidetodoc.com/presentation_image_h2/81def57c997796372a709fb5fc171f16/image-47.jpg)
![Direct electronics with AFP (additional positive feedback) [Drung] Direct electronics with AFP (additional positive feedback) [Drung]](https://slidetodoc.com/presentation_image_h2/81def57c997796372a709fb5fc171f16/image-48.jpg)
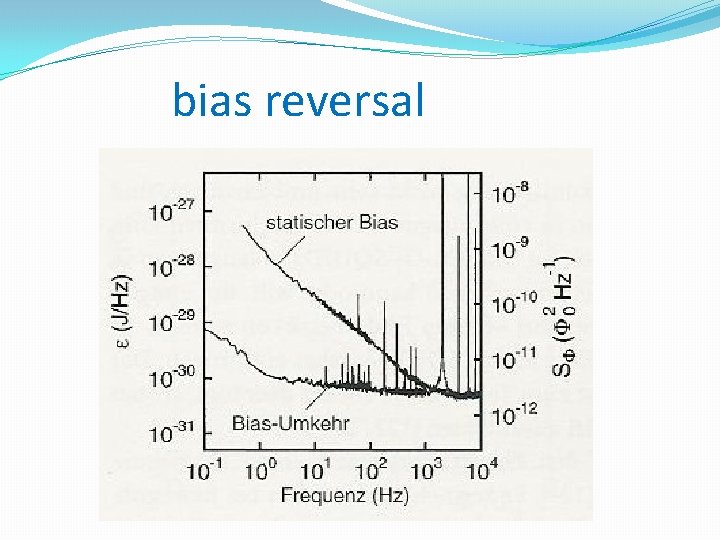
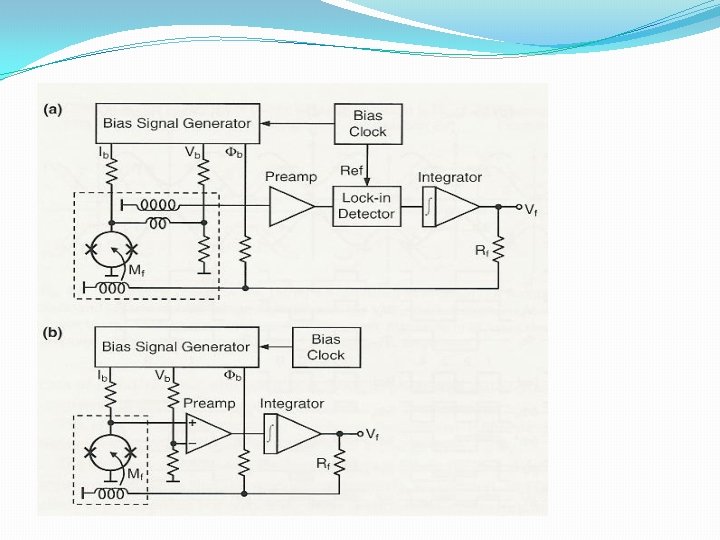
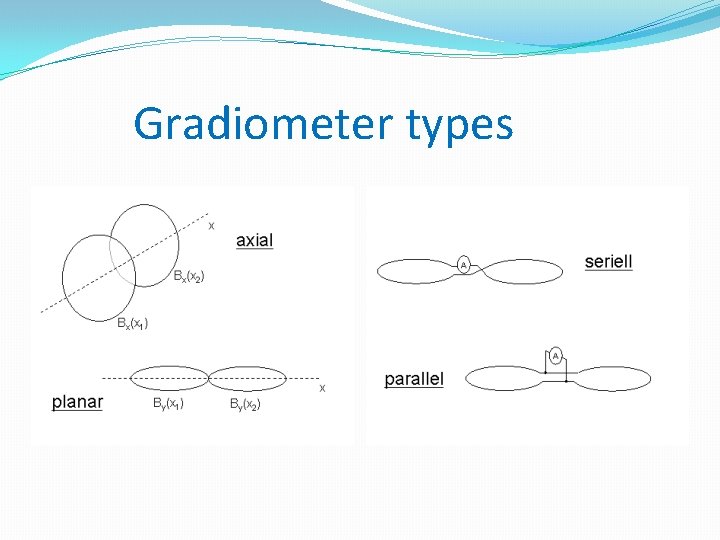
![Wire incoupling structures [ J. Clarke ] Wire incoupling structures [ J. Clarke ]](https://slidetodoc.com/presentation_image_h2/81def57c997796372a709fb5fc171f16/image-52.jpg)
![Multi channel gradiometer [ Biomagnetisches Zentrum der FSU Jena ] Multi channel gradiometer [ Biomagnetisches Zentrum der FSU Jena ]](https://slidetodoc.com/presentation_image_h2/81def57c997796372a709fb5fc171f16/image-53.jpg)
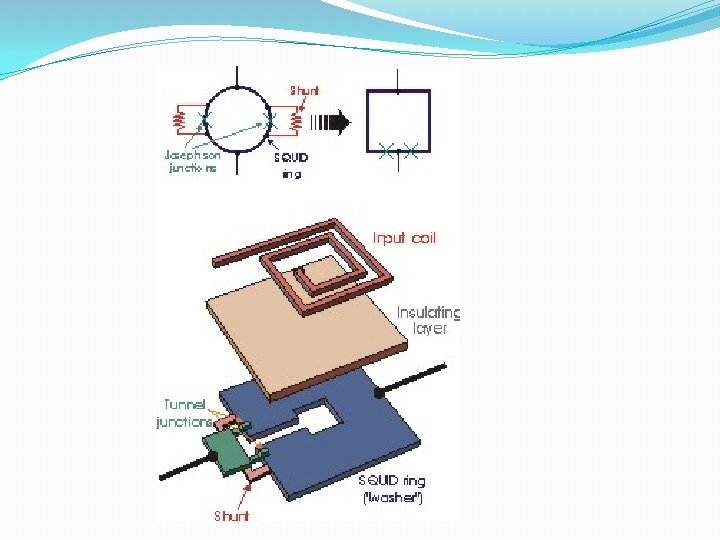
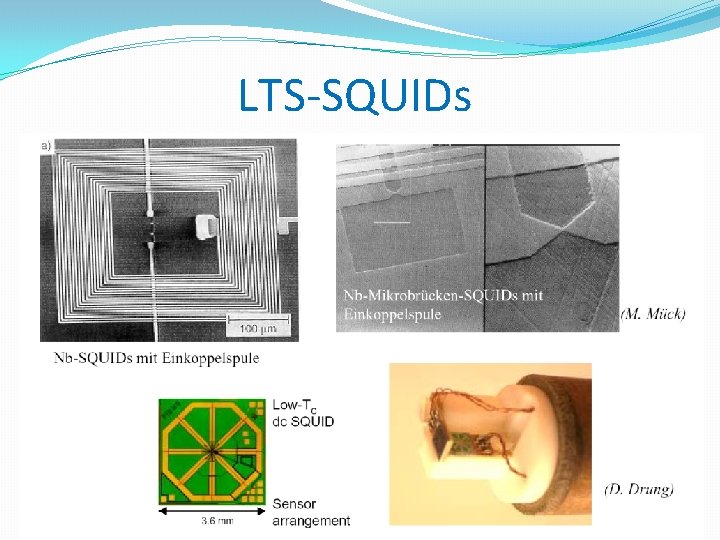
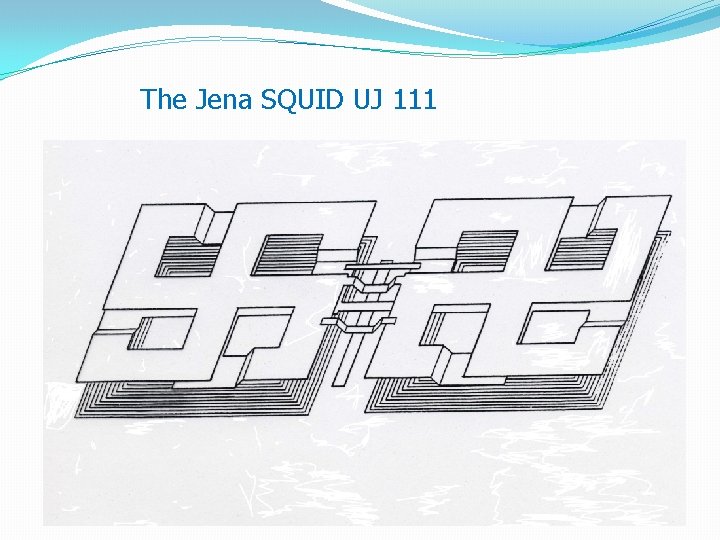
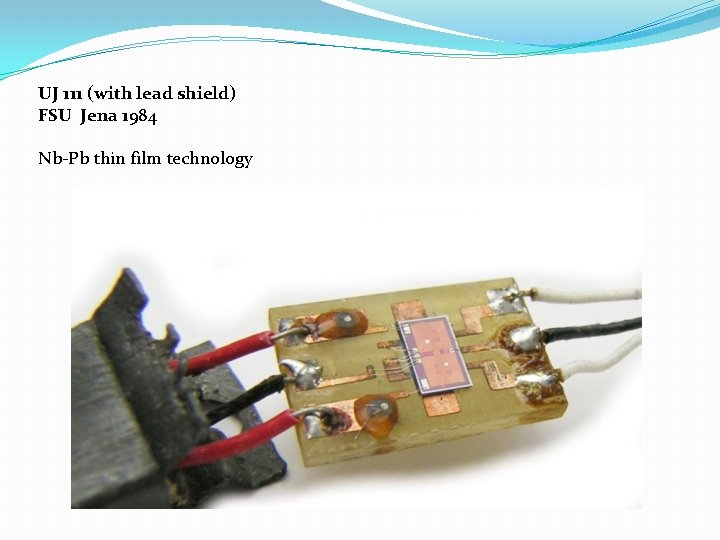
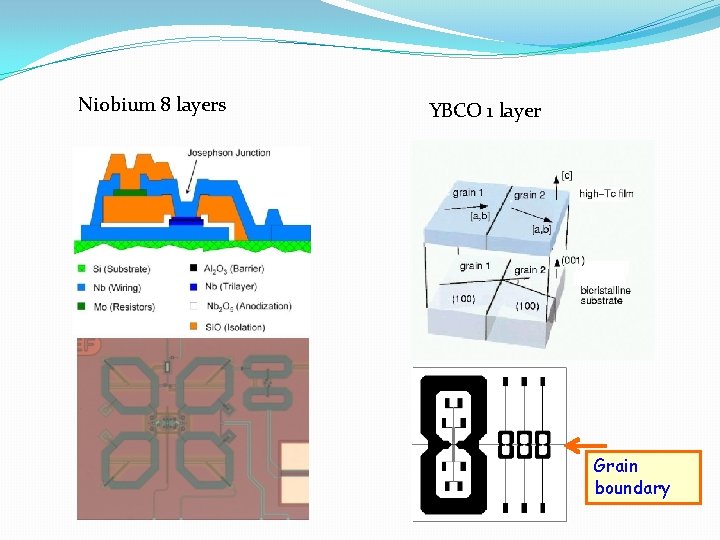
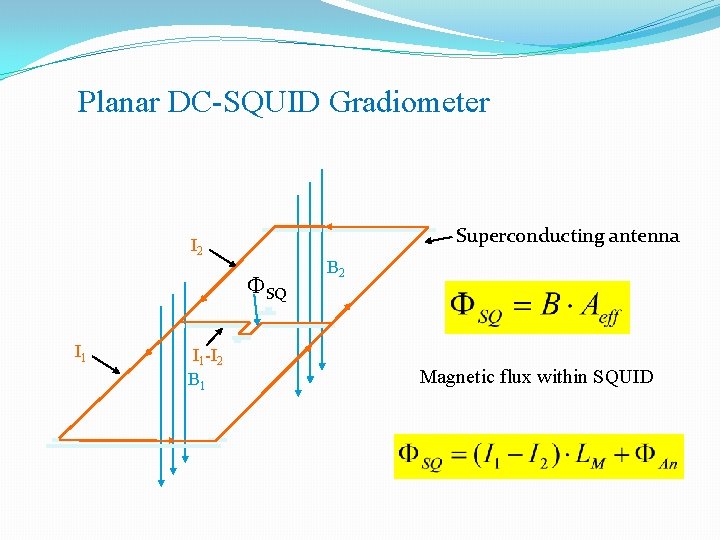
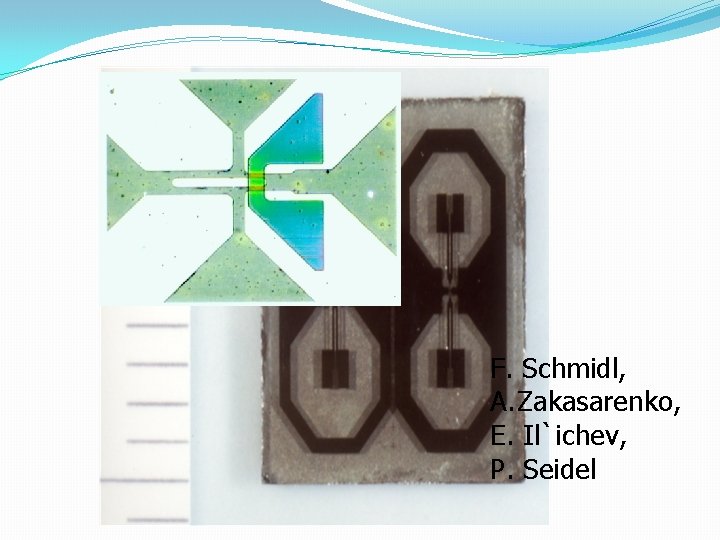
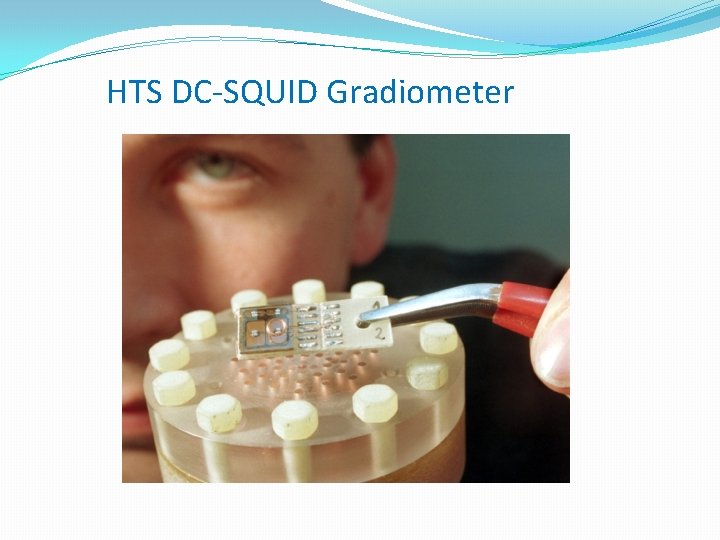
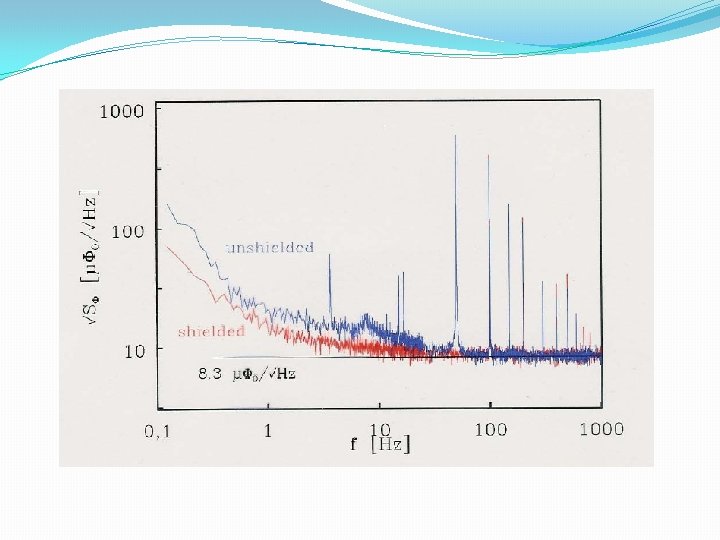
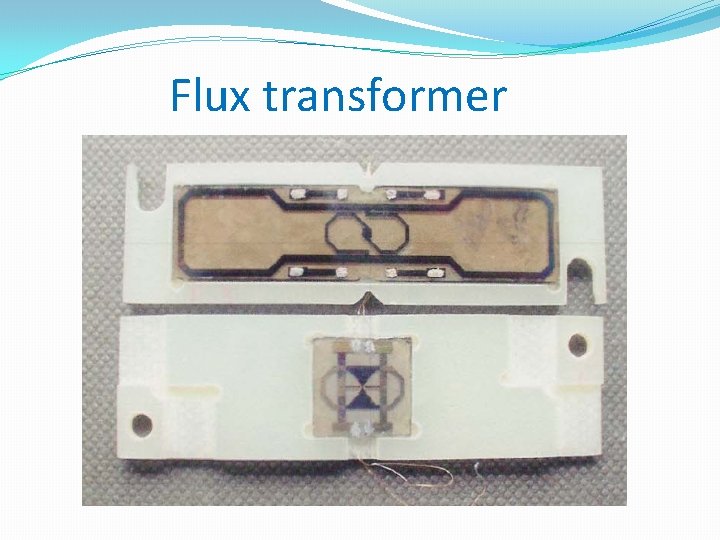
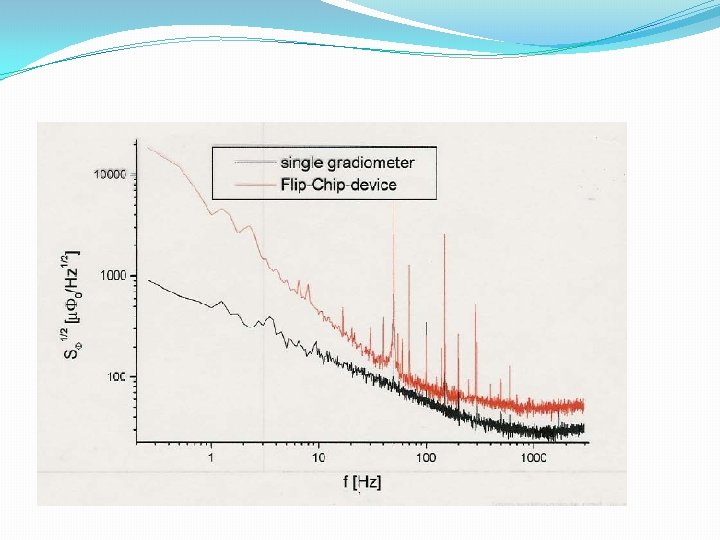
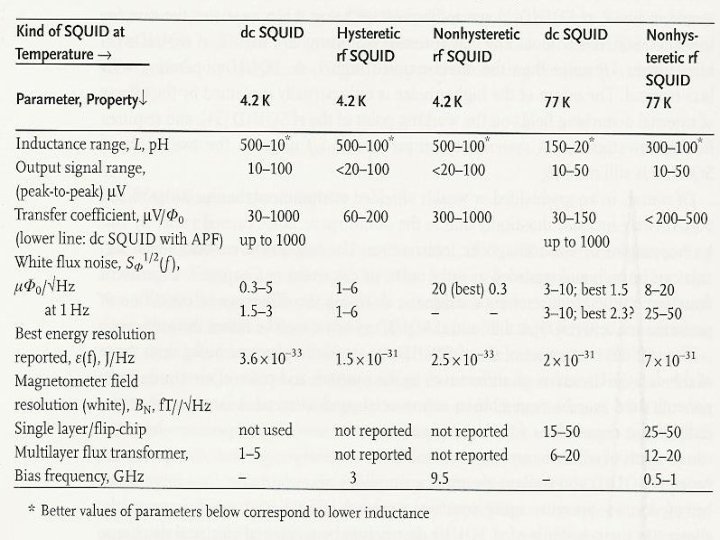
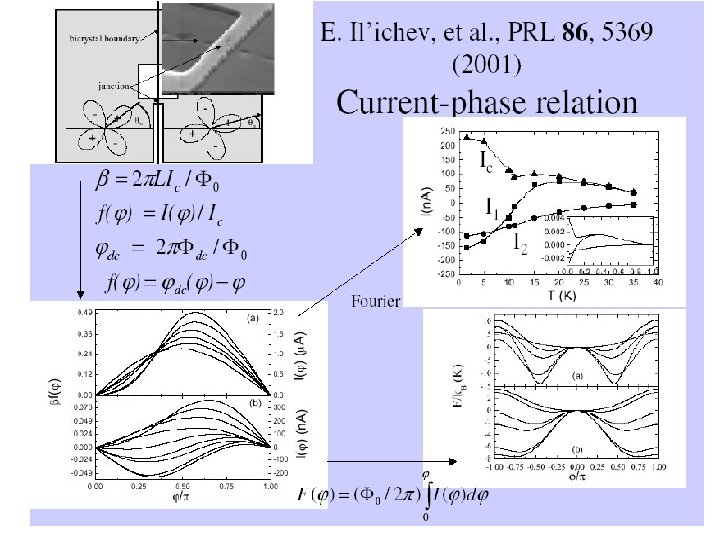
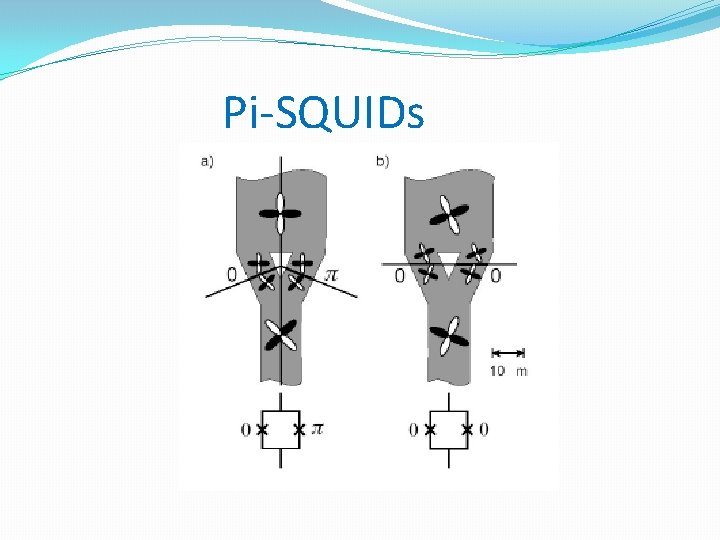
![[Chesca] [Chesca]](https://slidetodoc.com/presentation_image_h2/81def57c997796372a709fb5fc171f16/image-68.jpg)
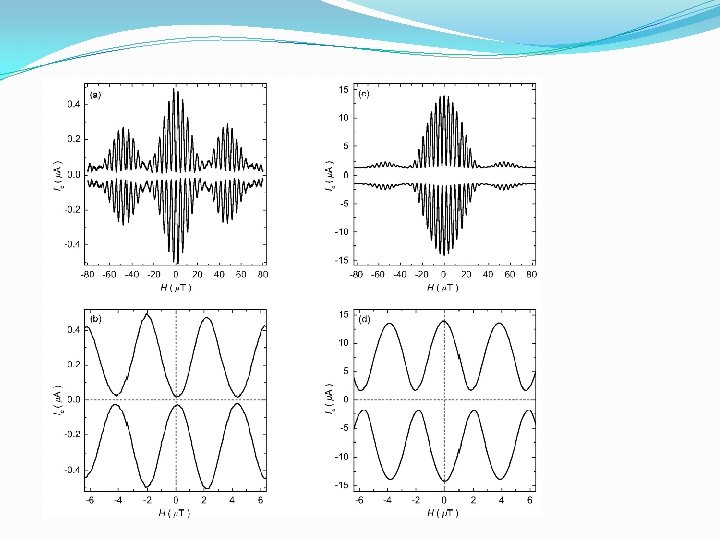
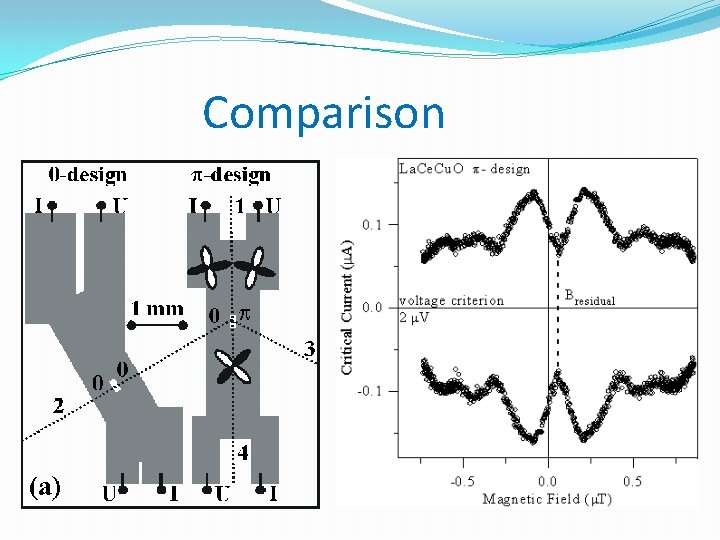
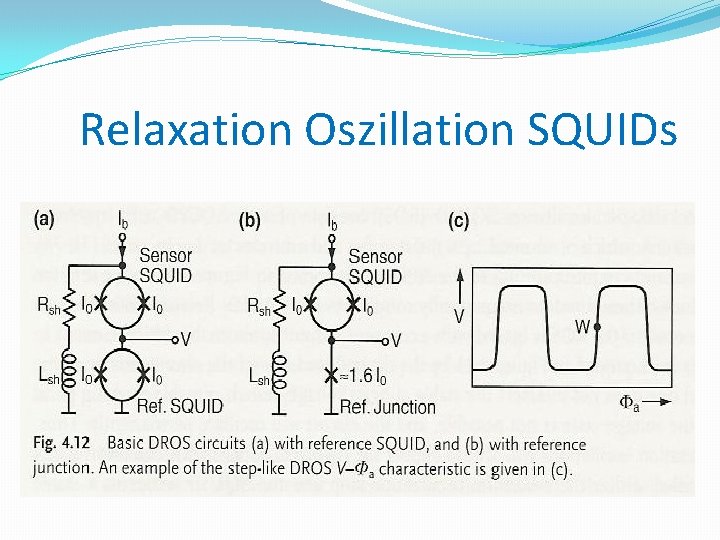
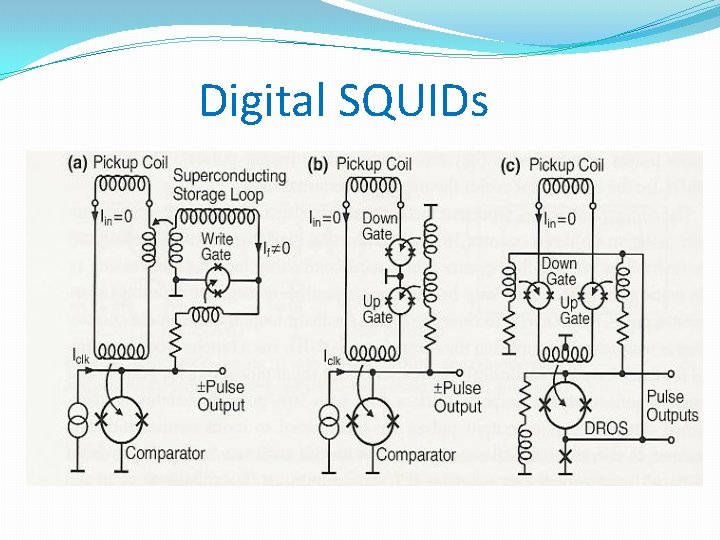
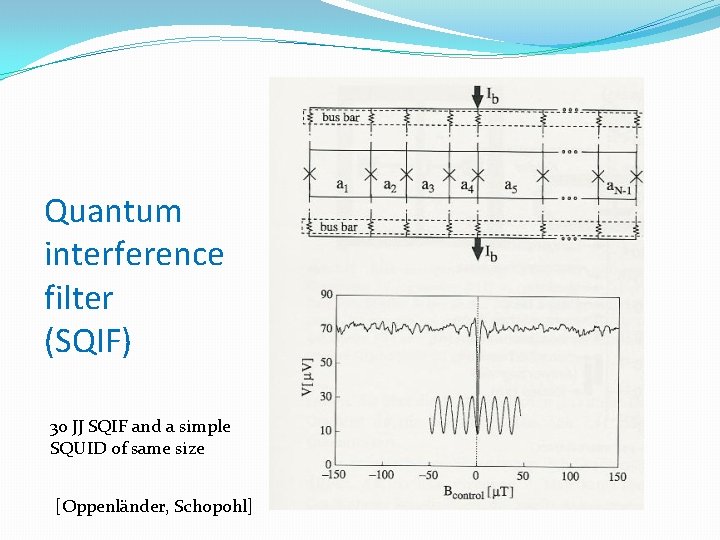
![Serial SQIF [Schultze] Serial SQIF [Schultze]](https://slidetodoc.com/presentation_image_h2/81def57c997796372a709fb5fc171f16/image-74.jpg)
![2 p. T / √ Hz [IPHT Jena]} 2 p. T / √ Hz [IPHT Jena]}](https://slidetodoc.com/presentation_image_h2/81def57c997796372a709fb5fc171f16/image-75.jpg)
![2 D- SQIF array [R. Fagaly] 2 D- SQIF array [R. Fagaly]](https://slidetodoc.com/presentation_image_h2/81def57c997796372a709fb5fc171f16/image-76.jpg)
![Bi-SQUID [R. Fagaly] Bi-SQUID [R. Fagaly]](https://slidetodoc.com/presentation_image_h2/81def57c997796372a709fb5fc171f16/image-77.jpg)
- Slides: 77
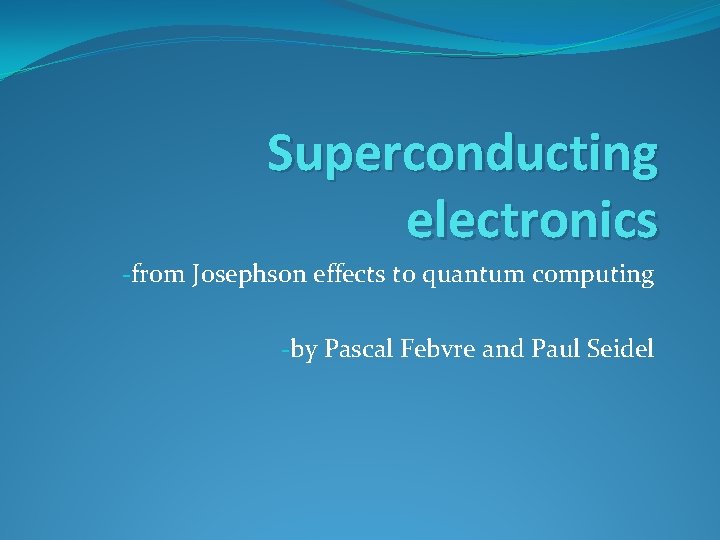
Superconducting electronics -from Josephson effects to quantum computing -by Pascal Febvre and Paul Seidel
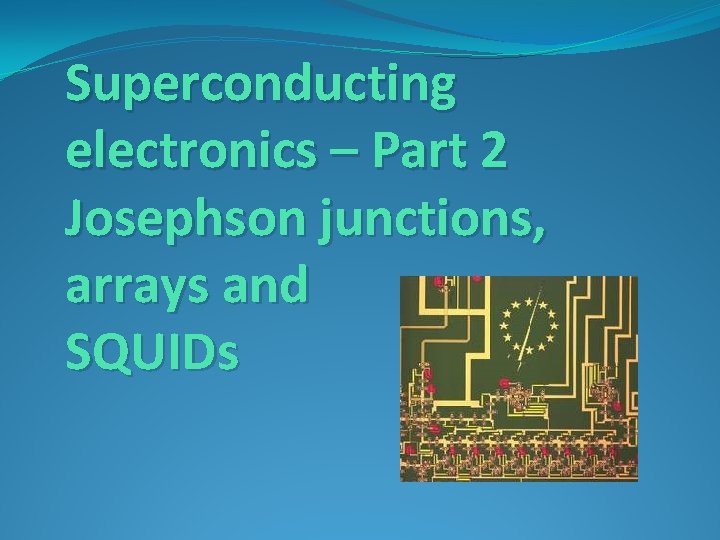
Superconducting electronics – Part 2 Josephson junctions, arrays and SQUIDs
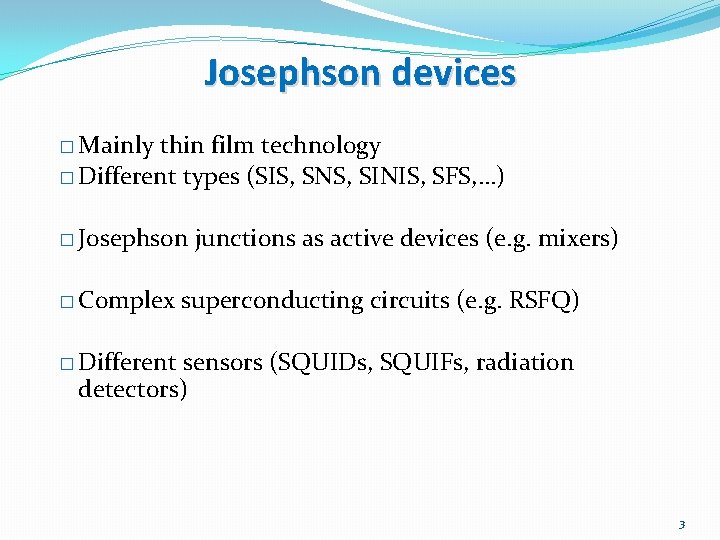
Josephson devices � Mainly thin film technology � Different types (SIS, SNS, SINIS, SFS, …) � Josephson � Complex junctions as active devices (e. g. mixers) superconducting circuits (e. g. RSFQ) � Different sensors (SQUIDs, SQUIFs, radiation detectors) 3
![Thin film preparation methods R Wördenweber Thin film preparation methods [R. Wördenweber]](https://slidetodoc.com/presentation_image_h2/81def57c997796372a709fb5fc171f16/image-4.jpg)
Thin film preparation methods [R. Wördenweber]
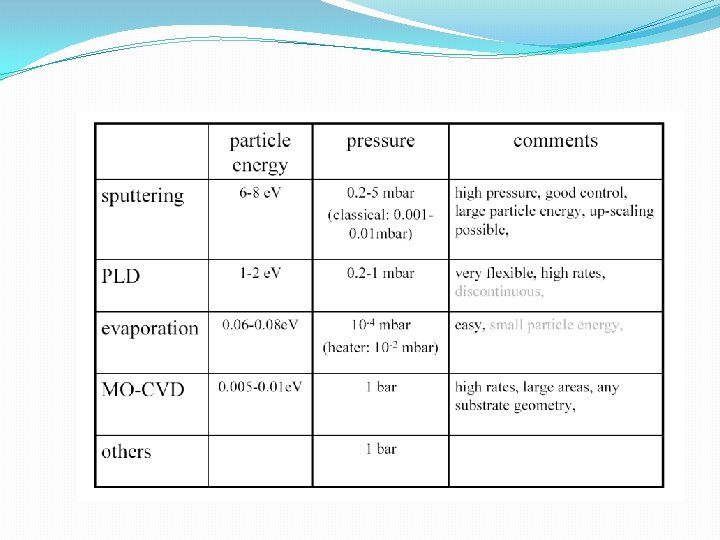
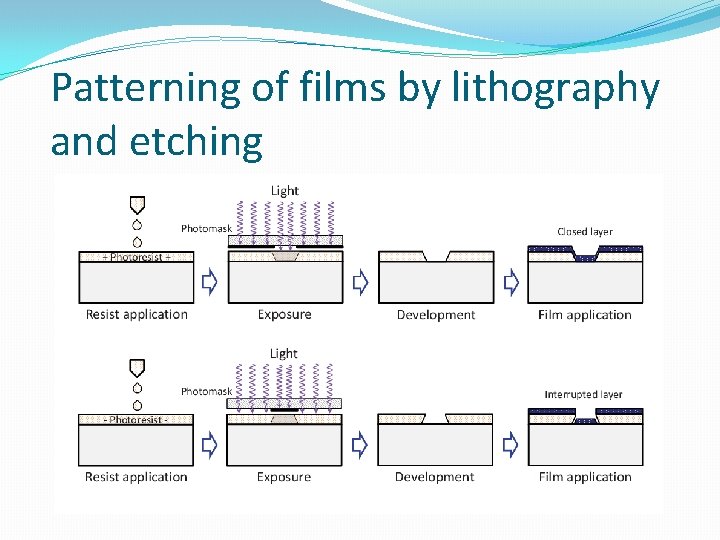
Patterning of films by lithography and etching
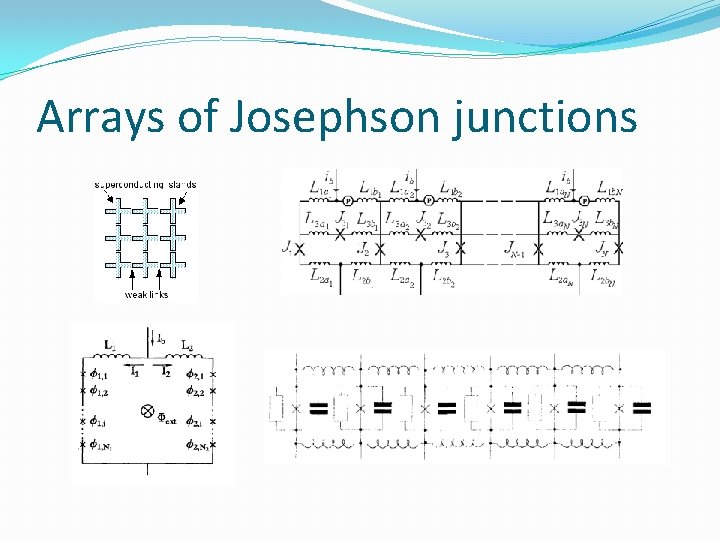
Arrays of Josephson junctions
![Serial arrays for voltage standard wikipedia Serial arrays for voltage standard [wikipedia]](https://slidetodoc.com/presentation_image_h2/81def57c997796372a709fb5fc171f16/image-8.jpg)
Serial arrays for voltage standard [wikipedia]
![J Niemeier 9 [ J. Niemeier ] 9](https://slidetodoc.com/presentation_image_h2/81def57c997796372a709fb5fc171f16/image-9.jpg)
[ J. Niemeier ] 9
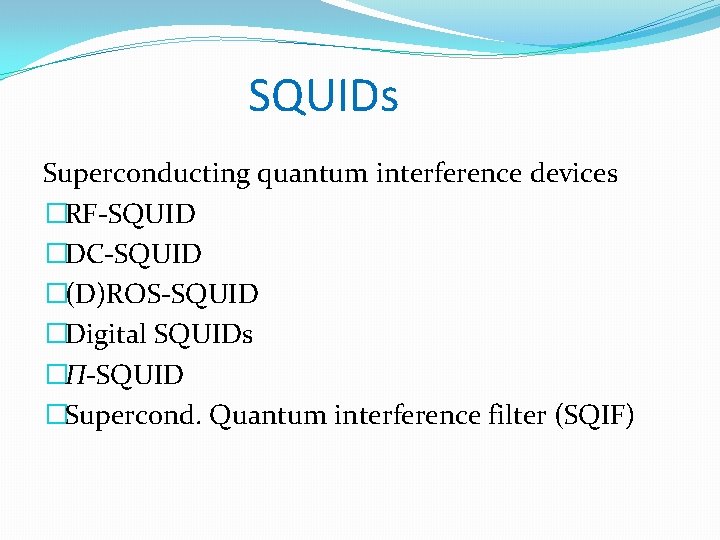
SQUIDs Superconducting quantum interference devices �RF-SQUID �DC-SQUID �(D)ROS-SQUID �Digital SQUIDs �Π-SQUID �Supercond. Quantum interference filter (SQIF)
![Gallop [Gallop]](https://slidetodoc.com/presentation_image_h2/81def57c997796372a709fb5fc171f16/image-11.jpg)
[Gallop]
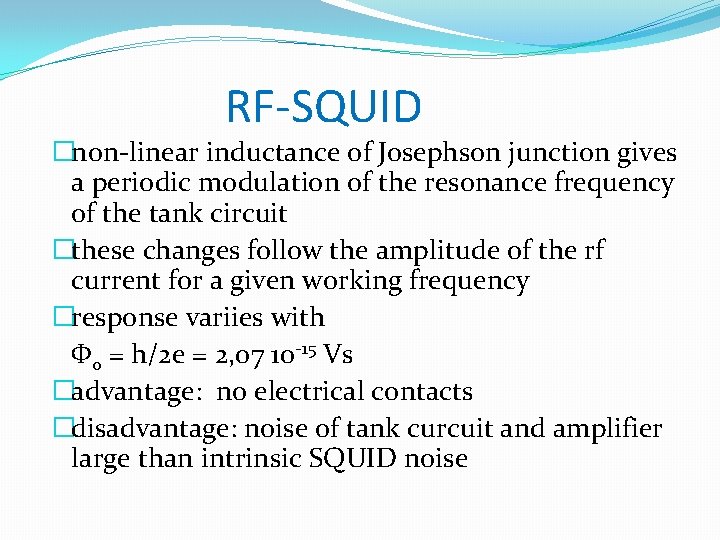
RF-SQUID �non-linear inductance of Josephson junction gives a periodic modulation of the resonance frequency of the tank circuit �these changes follow the amplitude of the rf current for a given working frequency �response variies with Φ 0 = h/2 e = 2, 07 10 -15 Vs �advantage: no electrical contacts �disadvantage: noise of tank curcuit and amplifier large than intrinsic SQUID noise
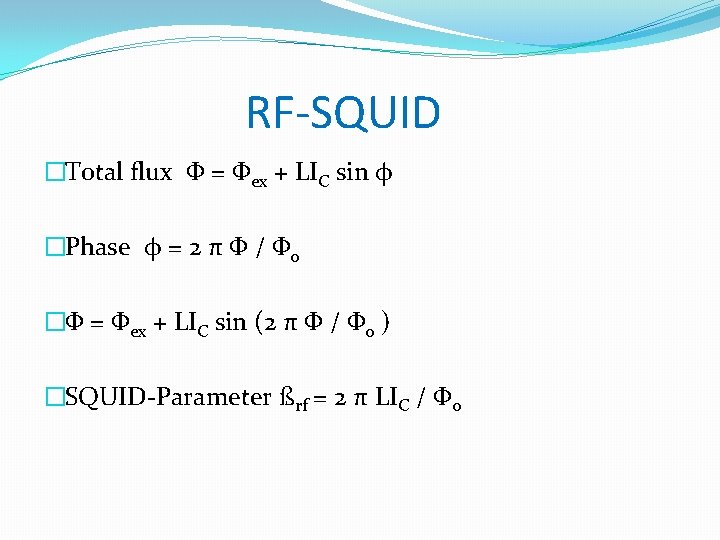
RF-SQUID �Total flux Φ = Φex + LIC sin φ �Phase φ = 2 π Φ / Φ 0 �Φ = Φex + LIC sin (2 π Φ / Φ 0 ) �SQUID-Parameter ßrf = 2 π LIC / Φ 0
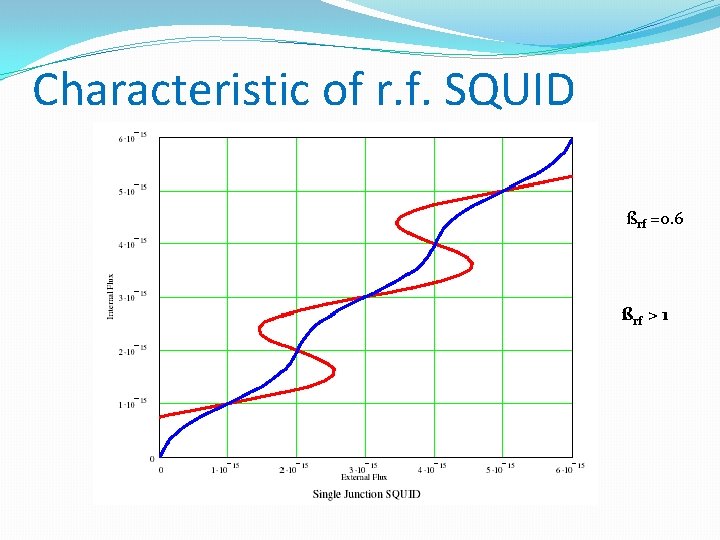
Characteristic of r. f. SQUID ßrf =0. 6 ßrf > 1
![LIC 1 25 Φ 0 k 0 k 1 Ruggiero Rudman LIC = 1. 25 Φ 0 k = 0 ↔ k =1 [Ruggiero, Rudman]](https://slidetodoc.com/presentation_image_h2/81def57c997796372a709fb5fc171f16/image-15.jpg)
LIC = 1. 25 Φ 0 k = 0 ↔ k =1 [Ruggiero, Rudman]
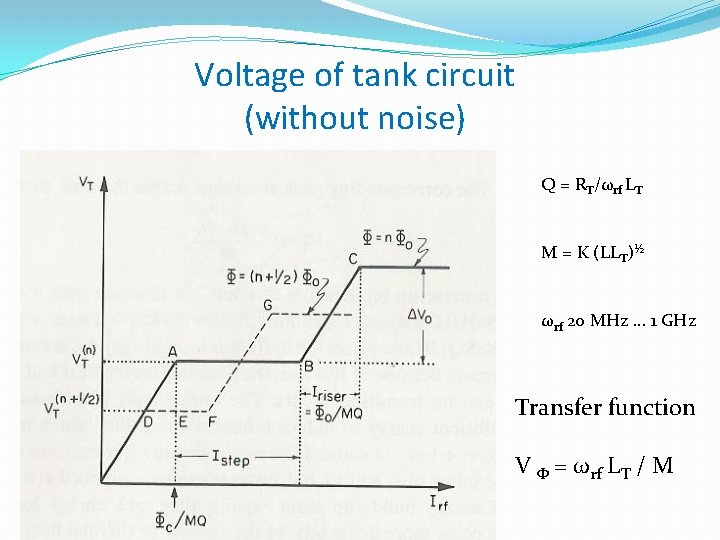
Voltage of tank circuit (without noise) Q = RT/ωrf LT M = K (LLT)½ ωrf 20 MHz … 1 GHz Transfer function V Φ = ωrf LT / M
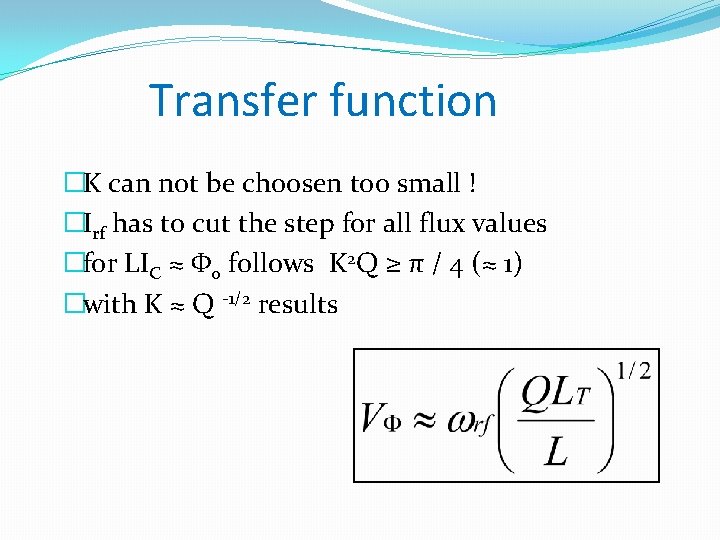
Transfer function �K can not be choosen too small ! �Irf has to cut the step for all flux values �for LIC ≈ Φ 0 follows K 2 Q ≥ π / 4 (≈ 1) �with K ≈ Q -1/2 results
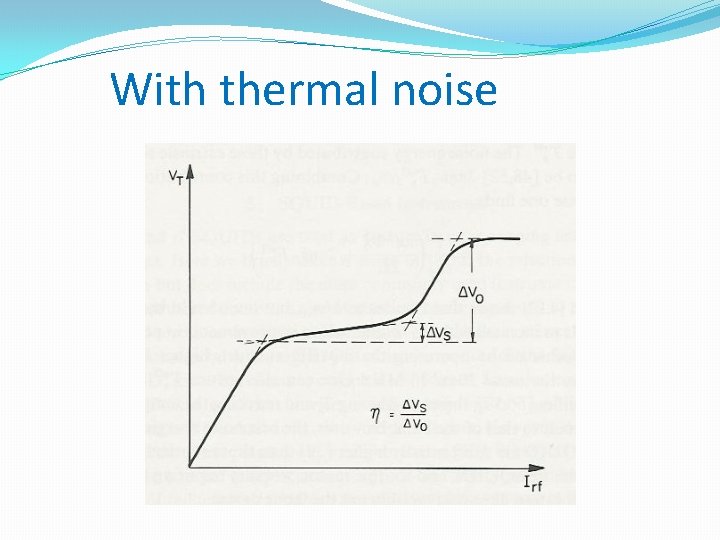
With thermal noise
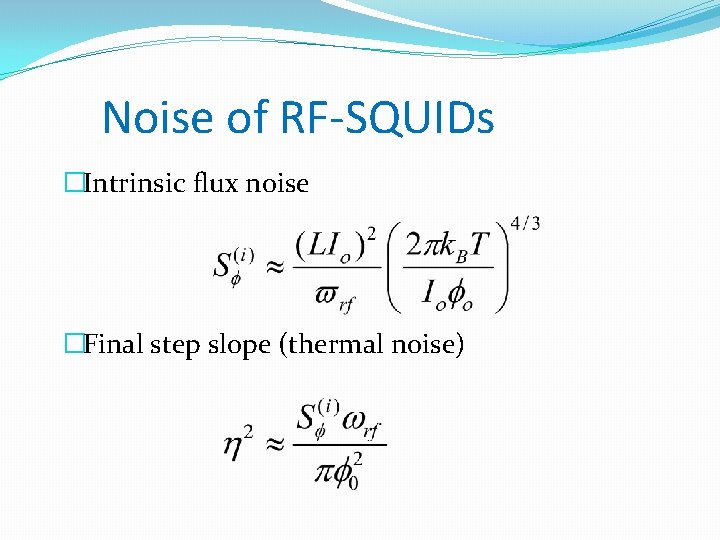
Noise of RF-SQUIDs �Intrinsic flux noise �Final step slope (thermal noise)
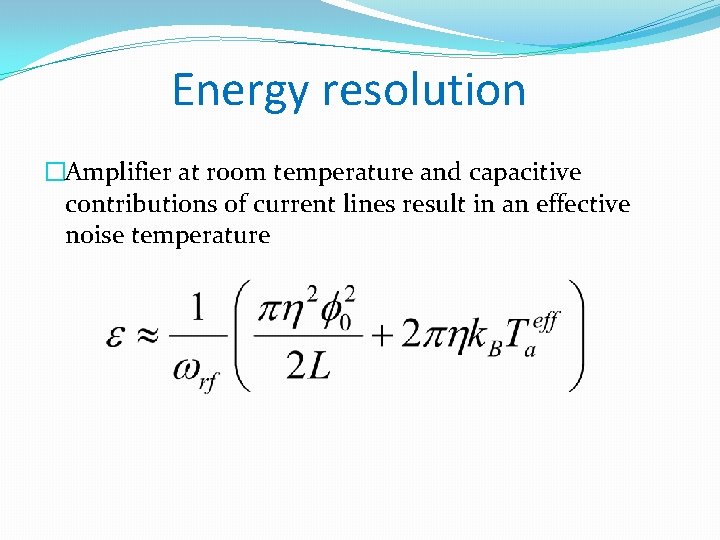
Energy resolution �Amplifier at room temperature and capacitive contributions of current lines result in an effective noise temperature
![Parameter SQUID Handbook Parameter [SQUID Handbook]](https://slidetodoc.com/presentation_image_h2/81def57c997796372a709fb5fc171f16/image-21.jpg)
Parameter [SQUID Handbook]
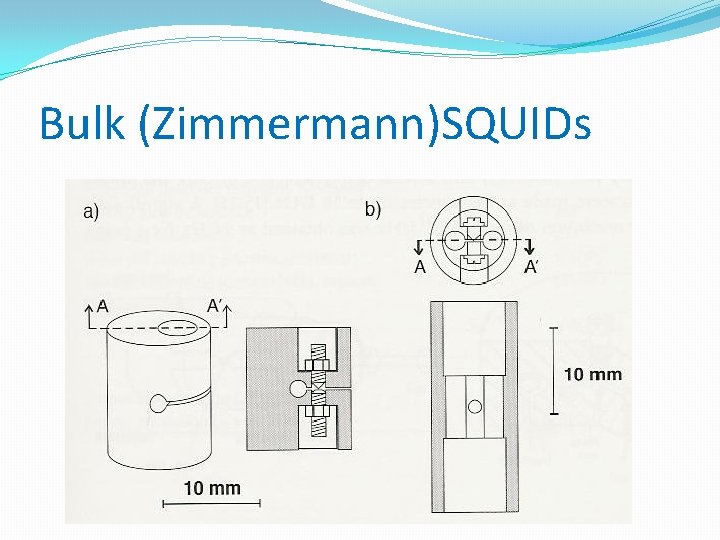
Bulk (Zimmermann)SQUIDs
![Transition to films SQUID Handbook Transition to films [SQUID Handbook]](https://slidetodoc.com/presentation_image_h2/81def57c997796372a709fb5fc171f16/image-23.jpg)
Transition to films [SQUID Handbook]
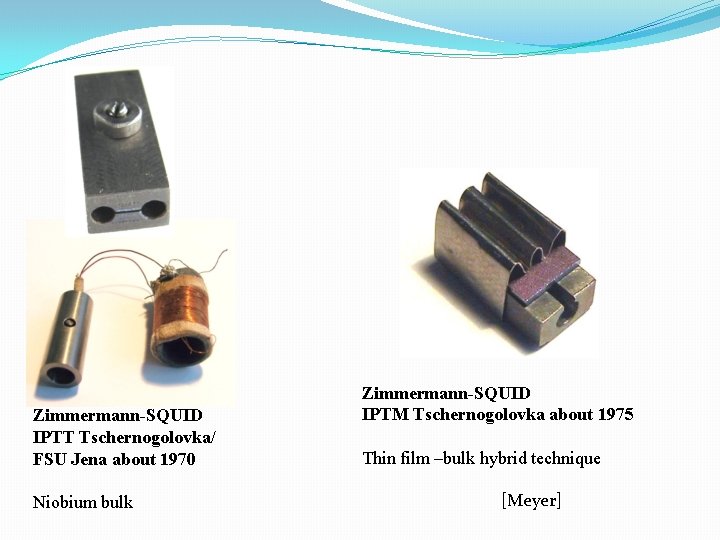
Zimmermann-SQUID IPTT Tschernogolovka/ FSU Jena about 1970 Niobium bulk Zimmermann-SQUID IPTM Tschernogolovka about 1975 Thin film –bulk hybrid technique [Meyer]
![HTSRFSQUID FZ Jülich HTS-RF-SQUID [FZ Jülich]](https://slidetodoc.com/presentation_image_h2/81def57c997796372a709fb5fc171f16/image-25.jpg)
HTS-RF-SQUID [FZ Jülich]
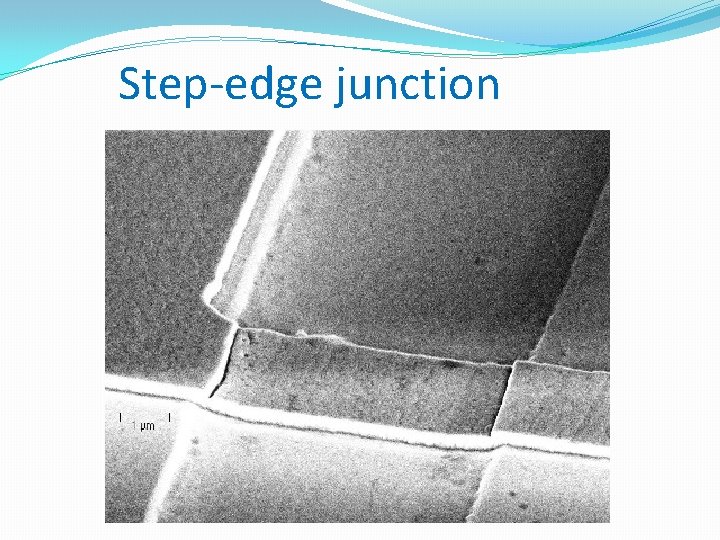
Step-edge junction
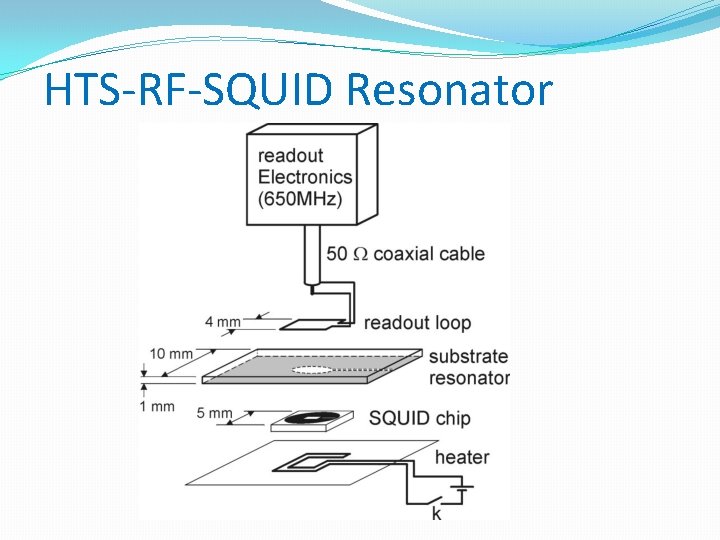
HTS-RF-SQUID Resonator
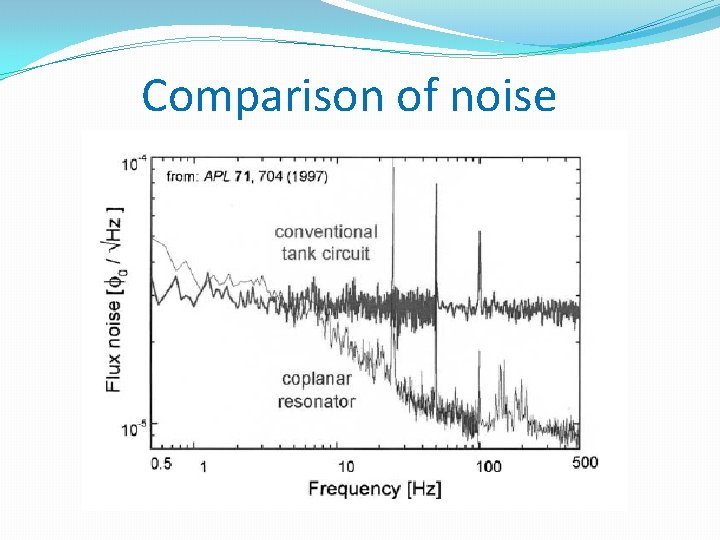
Comparison of noise
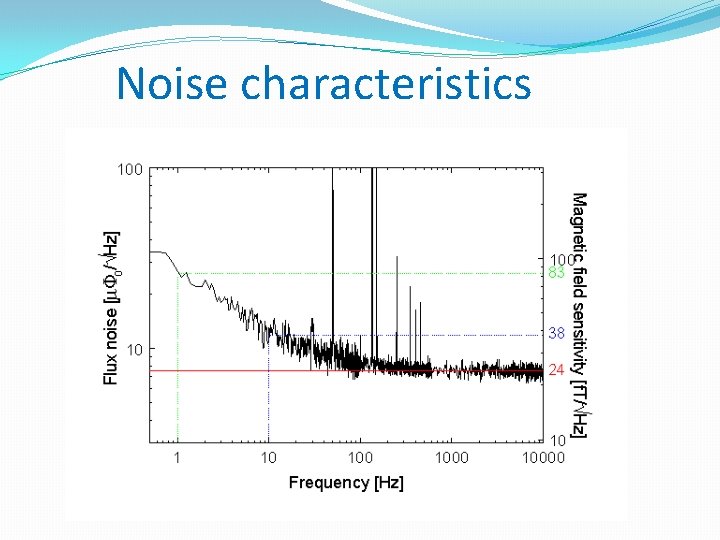
Noise characteristics
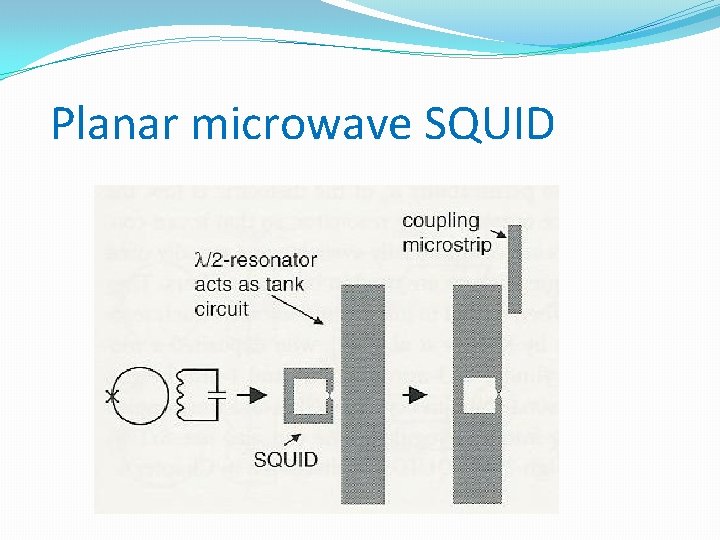
Planar microwave SQUID
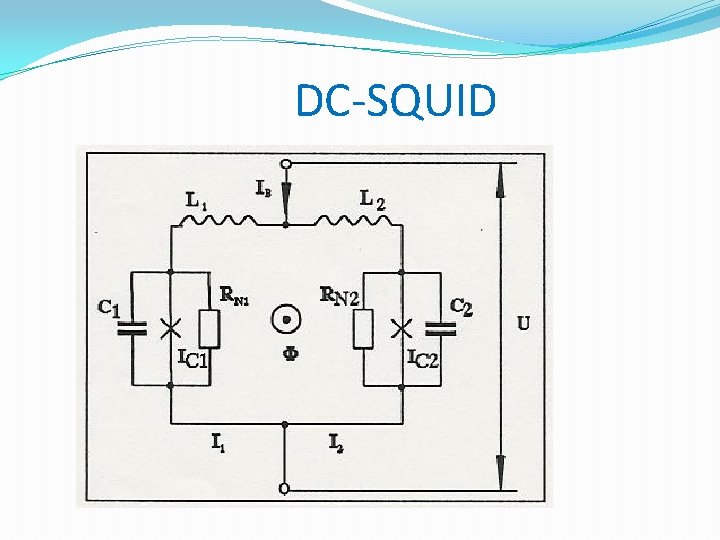
DC-SQUID
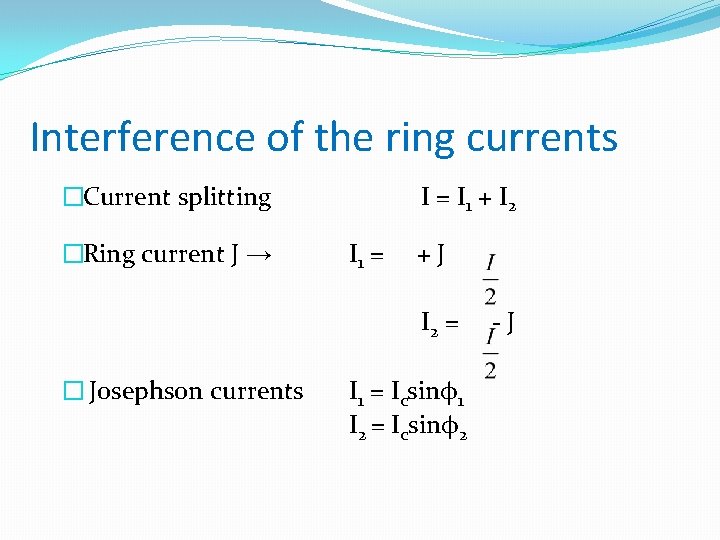
Interference of the ring currents �Current splitting �Ring current J → I = I 1 + I 2 I 1 = +J I 2 = � Josephson currents I 1 = Icsinφ1 I 2 = Icsinφ2 -J
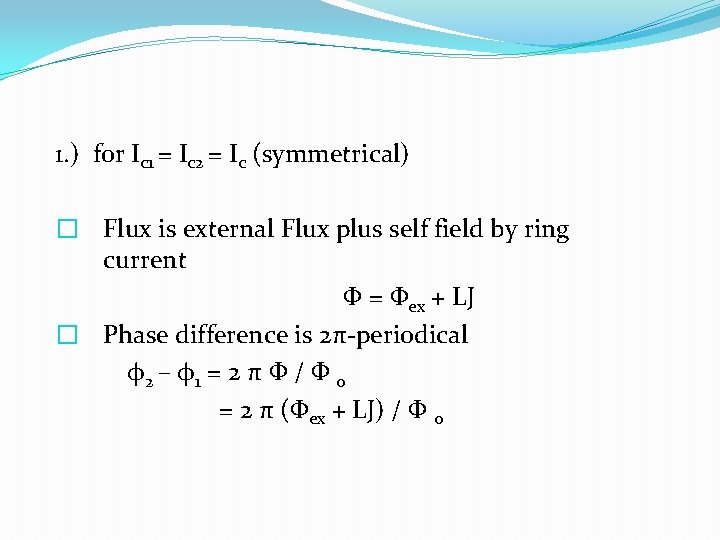
1. ) for Ic 1 = Ic 2 = Ic (symmetrical) � Flux is external Flux plus self field by ring current Φ = Φex + LJ � Phase difference is 2π-periodical φ2 – φ1 = 2 π Φ / Φ 0 = 2 π (Φex + LJ) / Φ 0
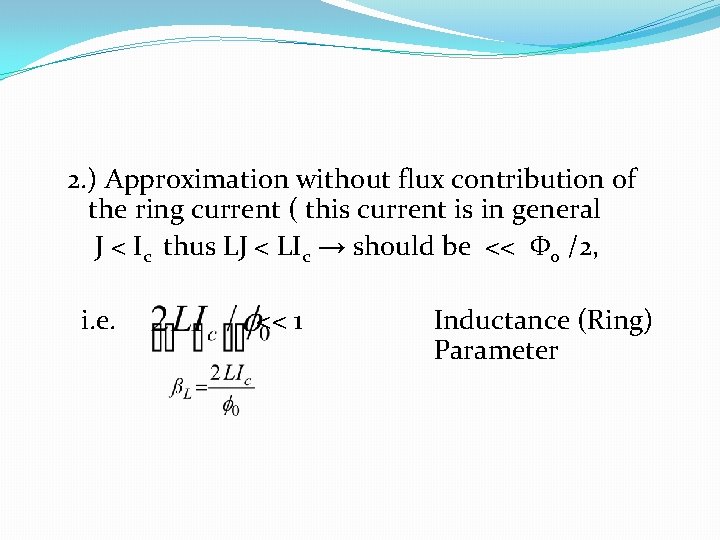
2. ) Approximation without flux contribution of the ring current ( this current is in general J < Ic thus LJ < LIc → should be << Φ 0 /2, i. e. << 1 Inductance (Ring) Parameter
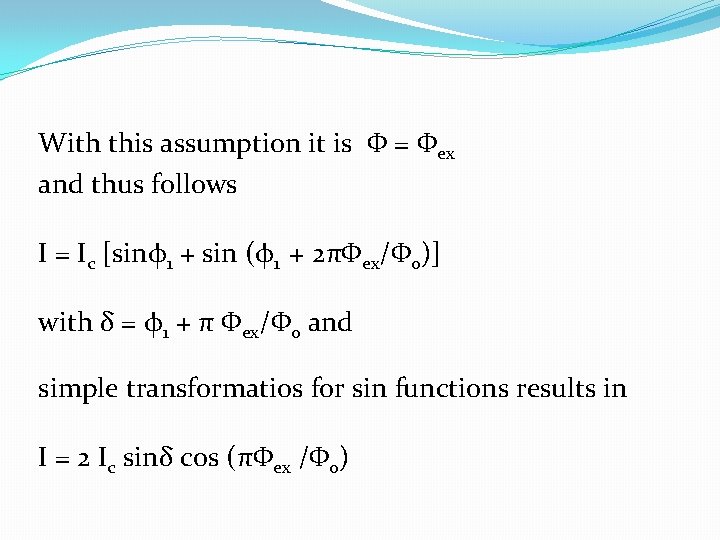
With this assumption it is Φ = Φex and thus follows I = Ic [sinφ1 + sin (φ1 + 2πΦex/Φ 0)] with δ = φ1 + π Φex/Φ 0 and simple transformatios for sin functions results in I = 2 Ic sinδ cos (πΦex /Φ 0)
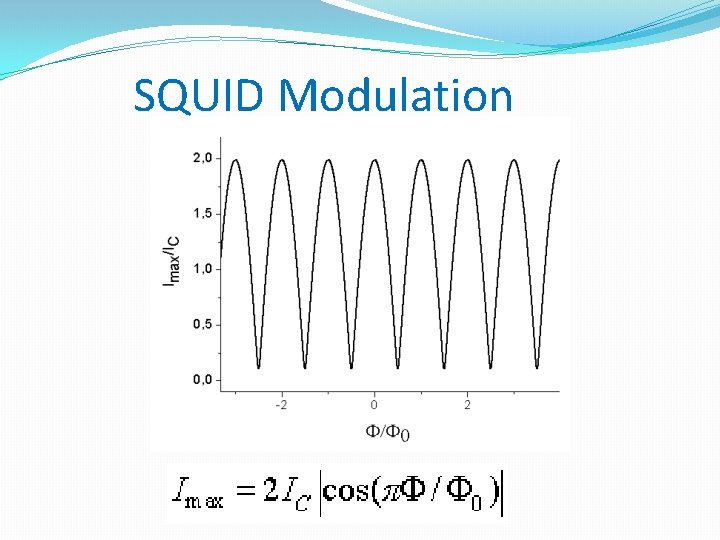
SQUID Modulation
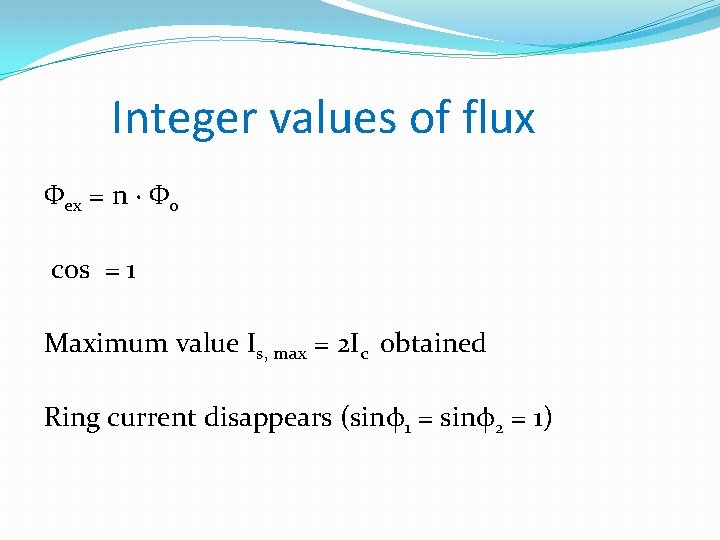
Integer values of flux Φex = n · Φ 0 cos = 1 Maximum value Is, max = 2 Ic obtained Ring current disappears (sinφ1 = sinφ2 = 1)
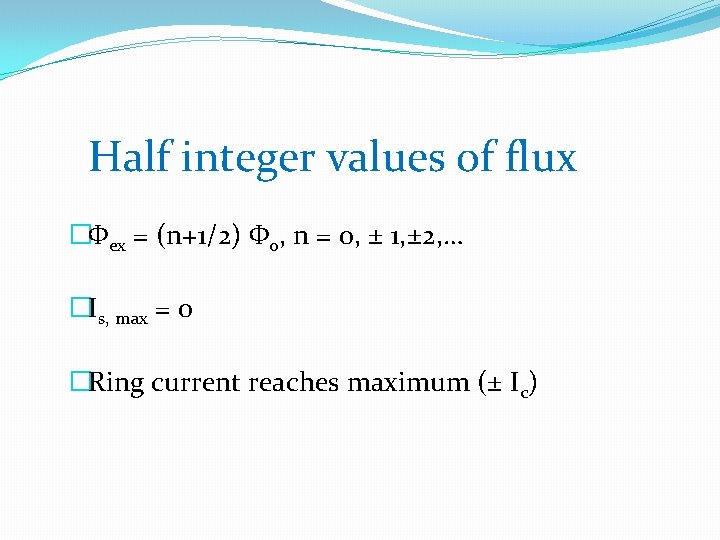
Half integer values of flux �Φex = (n+1/2) Φ 0, n = 0, ± 1, ± 2, … �Is, max = 0 �Ring current reaches maximum (± Ic)
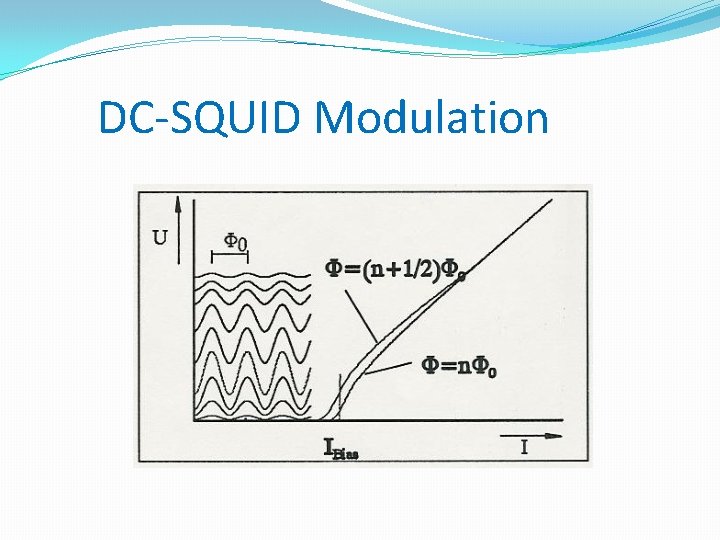
DC-SQUID Modulation
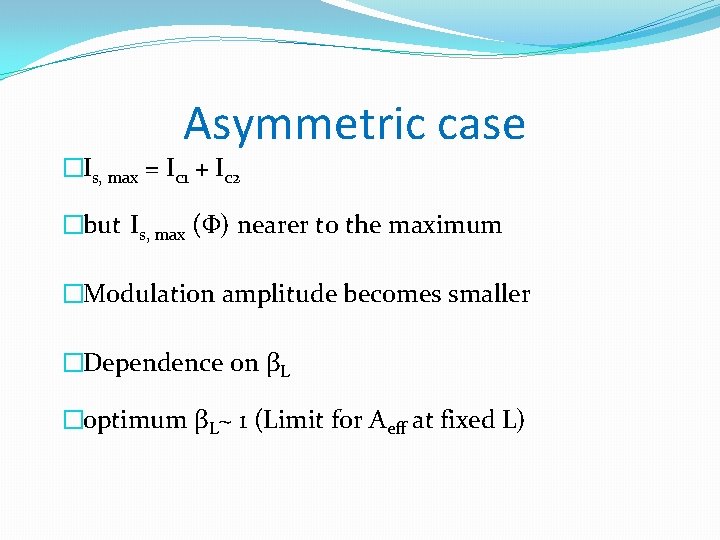
Asymmetric case �Is, max = Ic 1 + Ic 2 �but Is, max (Φ) nearer to the maximum �Modulation amplitude becomes smaller �Dependence on βL �optimum βL~ 1 (Limit for Aeff at fixed L)
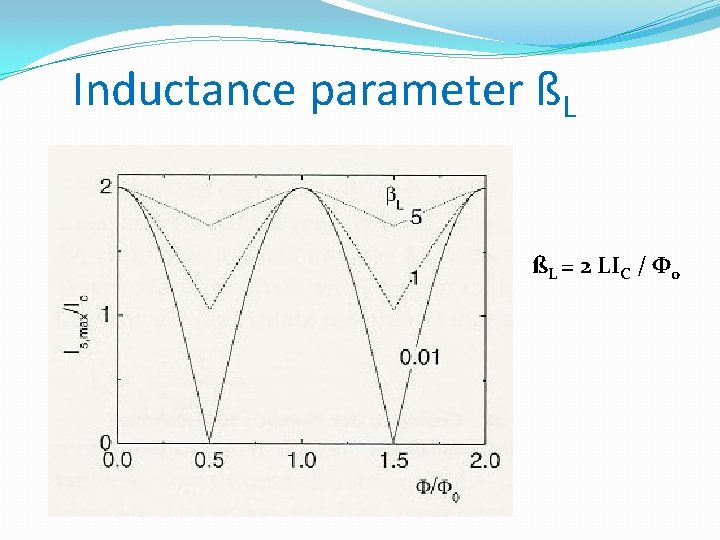
Inductance parameter ßL ßL = 2 LIC / Φ 0
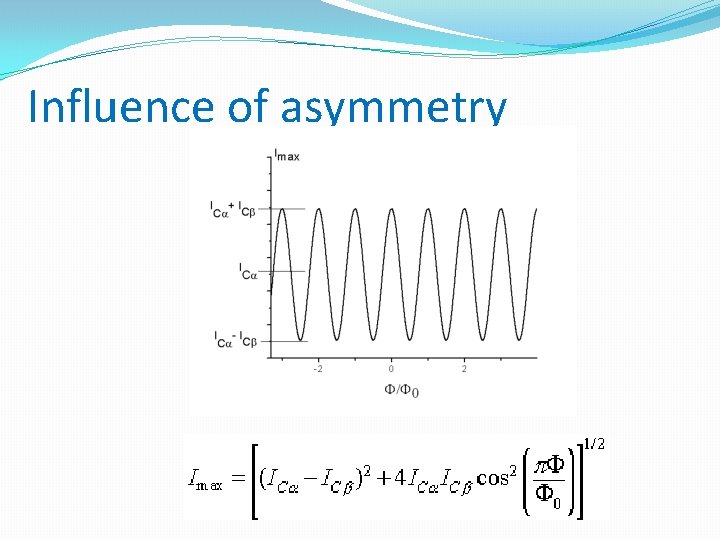
Influence of asymmetry
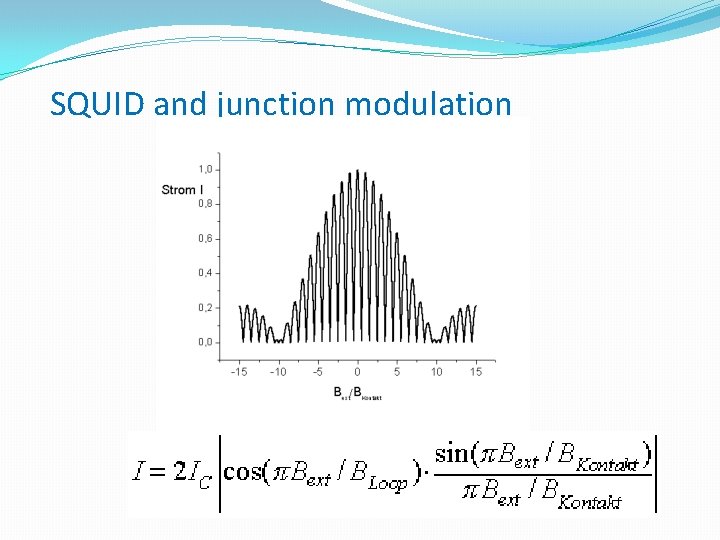
SQUID and junction modulation
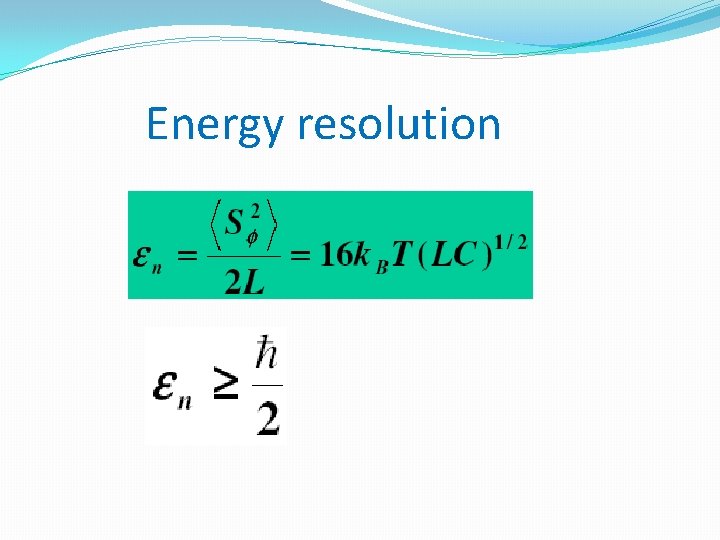
Energy resolution
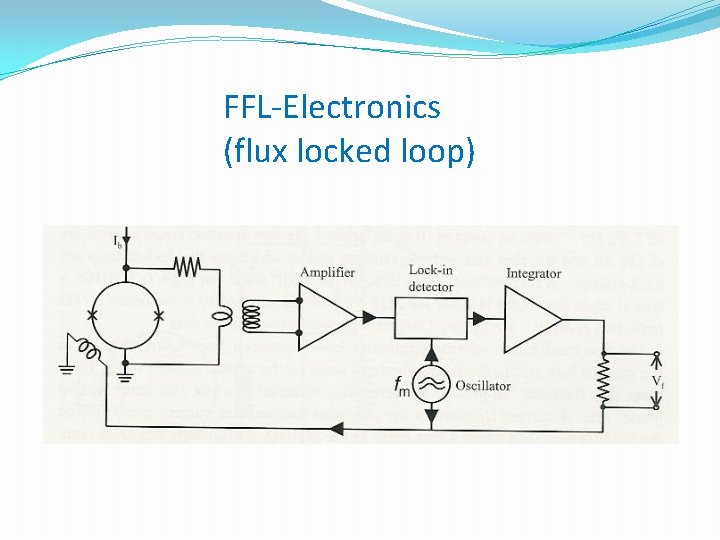
FFL-Electronics (flux locked loop)
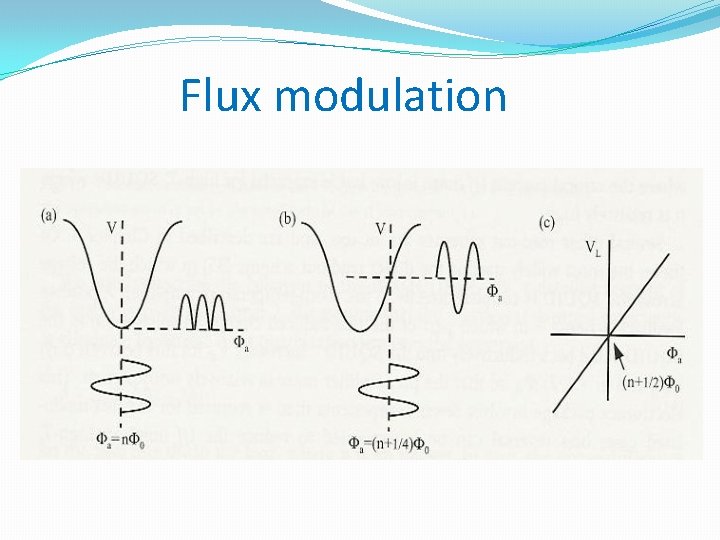
Flux modulation
![Drung [Drung]](https://slidetodoc.com/presentation_image_h2/81def57c997796372a709fb5fc171f16/image-47.jpg)
[Drung]
![Direct electronics with AFP additional positive feedback Drung Direct electronics with AFP (additional positive feedback) [Drung]](https://slidetodoc.com/presentation_image_h2/81def57c997796372a709fb5fc171f16/image-48.jpg)
Direct electronics with AFP (additional positive feedback) [Drung]
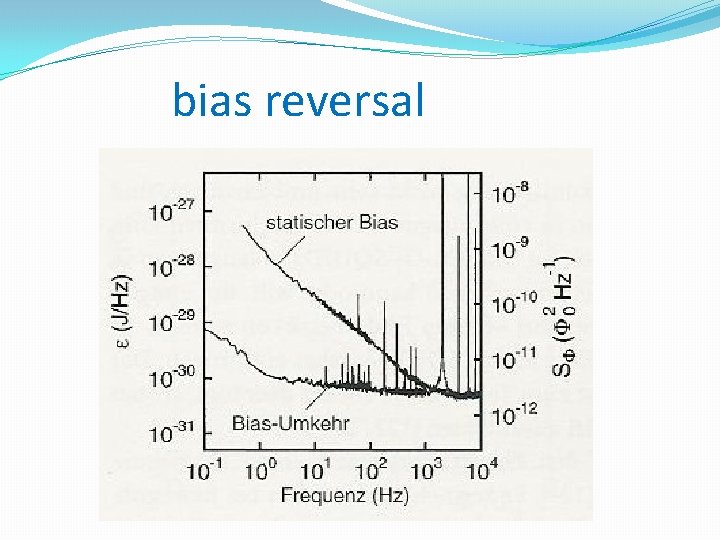
bias reversal
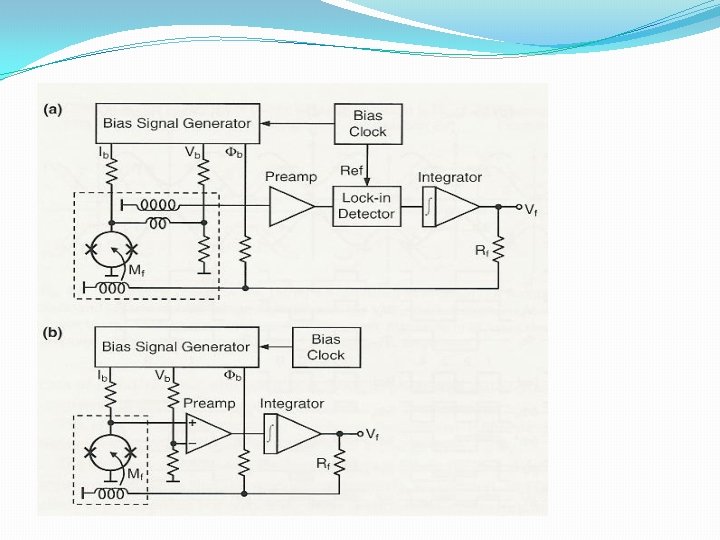
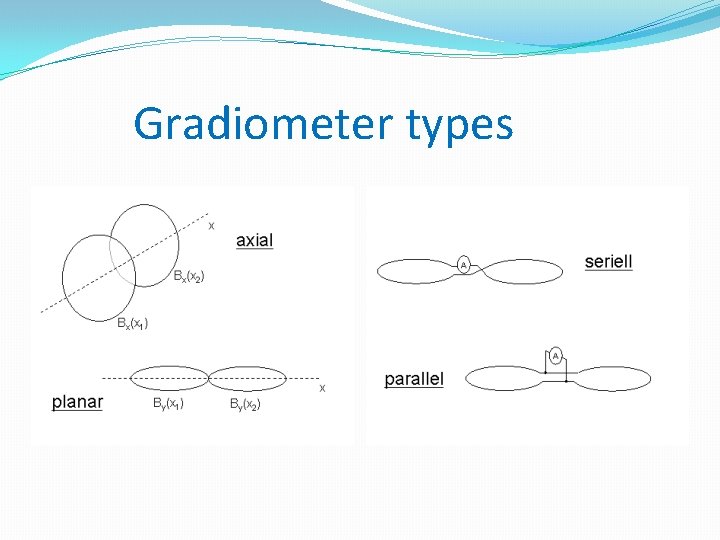
Gradiometer types
![Wire incoupling structures J Clarke Wire incoupling structures [ J. Clarke ]](https://slidetodoc.com/presentation_image_h2/81def57c997796372a709fb5fc171f16/image-52.jpg)
Wire incoupling structures [ J. Clarke ]
![Multi channel gradiometer Biomagnetisches Zentrum der FSU Jena Multi channel gradiometer [ Biomagnetisches Zentrum der FSU Jena ]](https://slidetodoc.com/presentation_image_h2/81def57c997796372a709fb5fc171f16/image-53.jpg)
Multi channel gradiometer [ Biomagnetisches Zentrum der FSU Jena ]
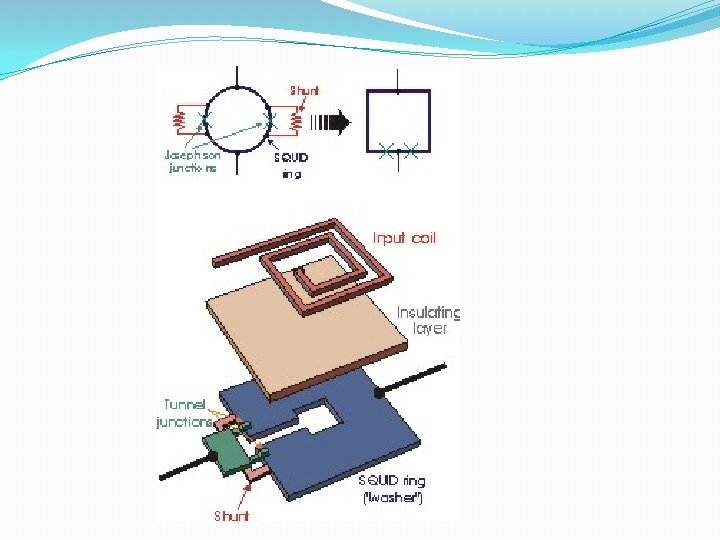
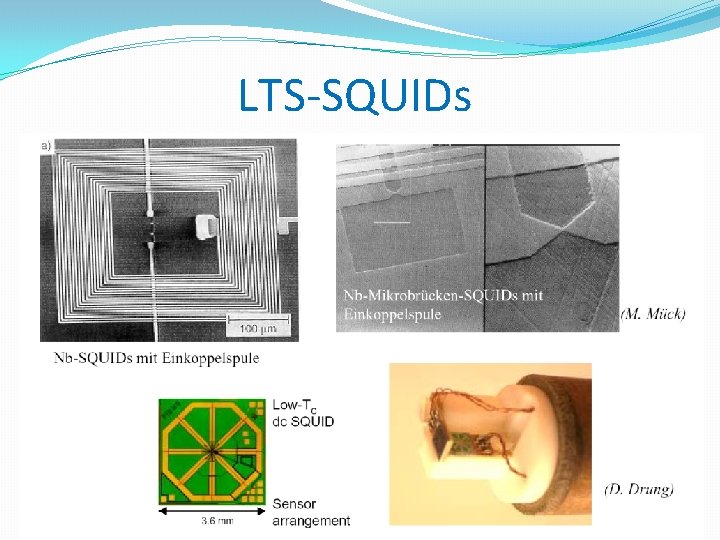
LTS-SQUIDs
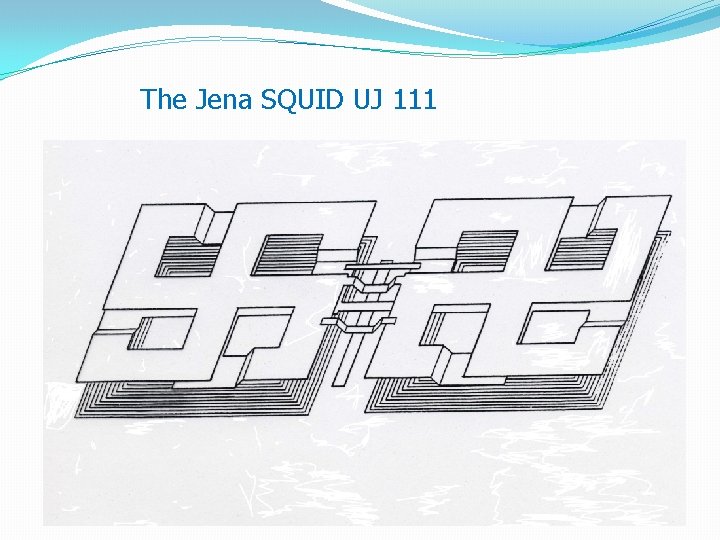
The Jena SQUID UJ 111
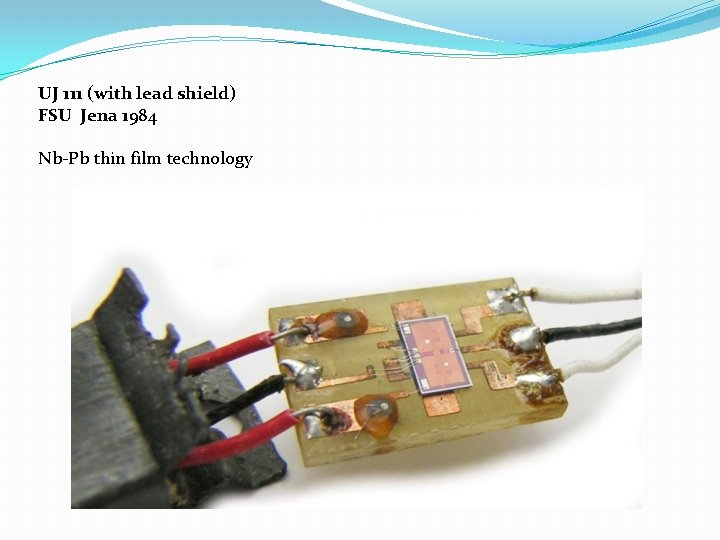
UJ 111 (with lead shield) FSU Jena 1984 Nb-Pb thin film technology
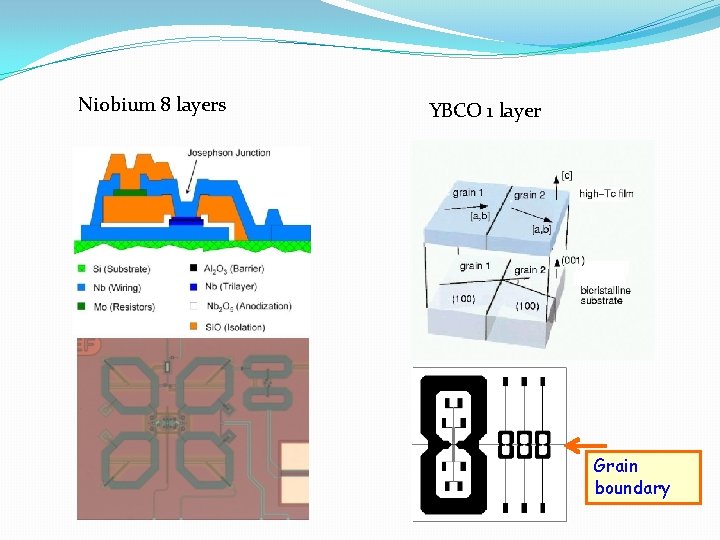
Niobium 8 layers YBCO 1 layer Grain boundary
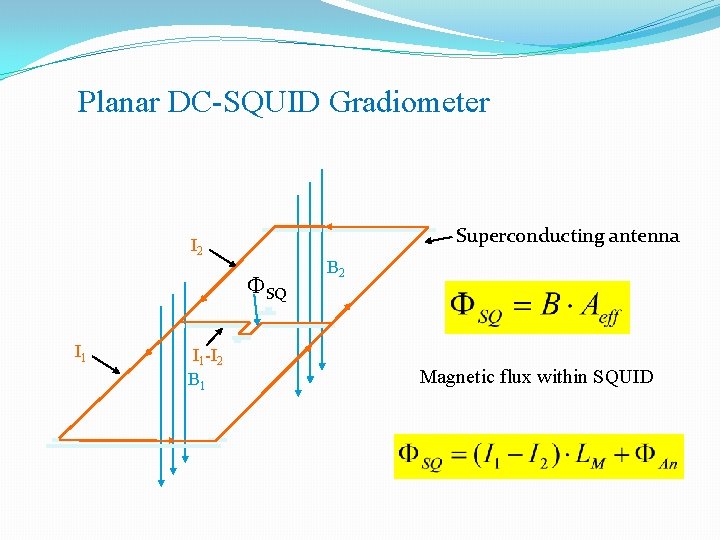
Planar DC-SQUID Gradiometer Superconducting antenna I 2 FSQ I 1 -I 2 B 1 B 2 Magnetic flux within SQUID
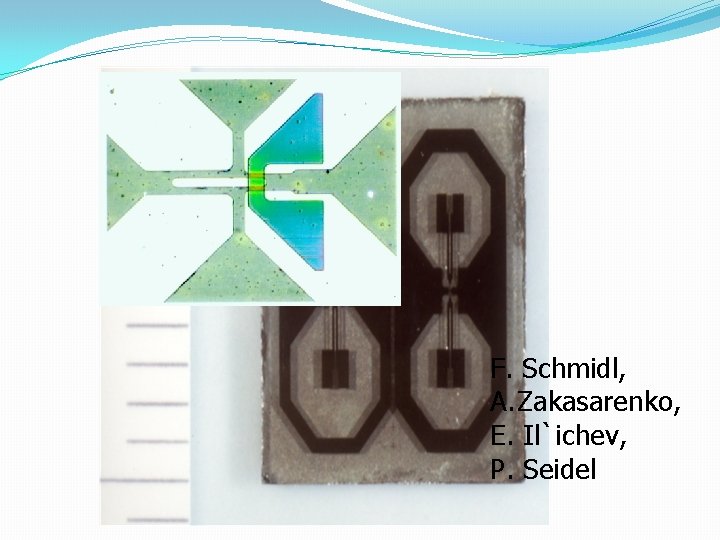
F. Schmidl, A. Zakasarenko, E. Il`ichev, P. Seidel
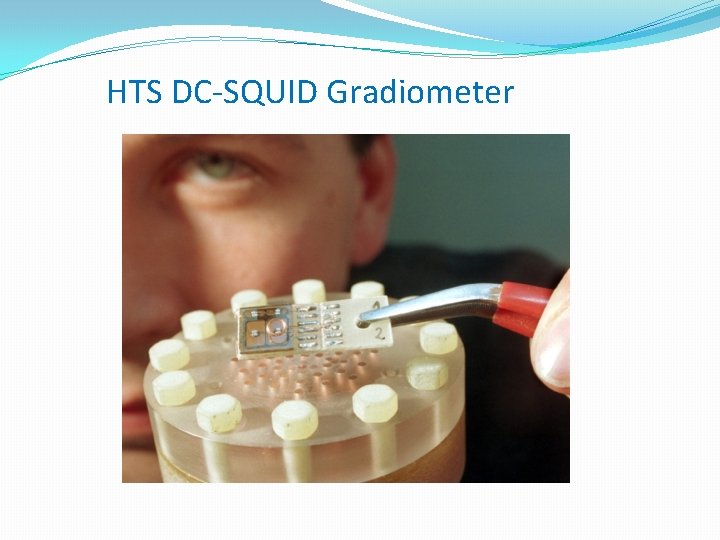
HTS DC-SQUID Gradiometer
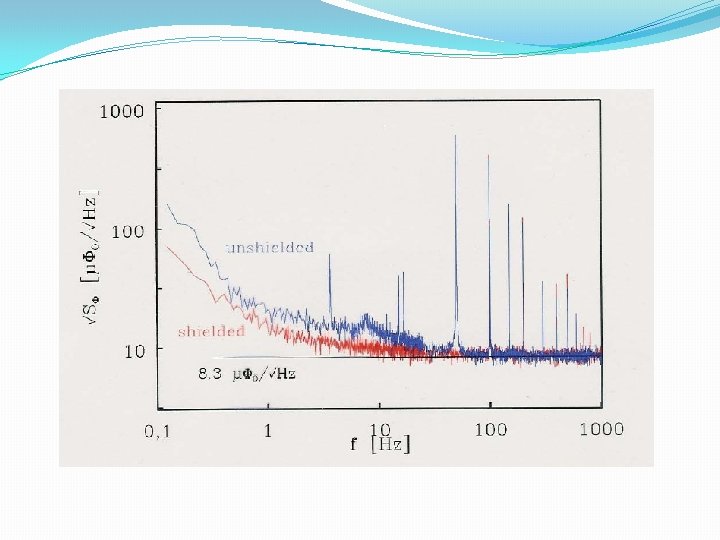
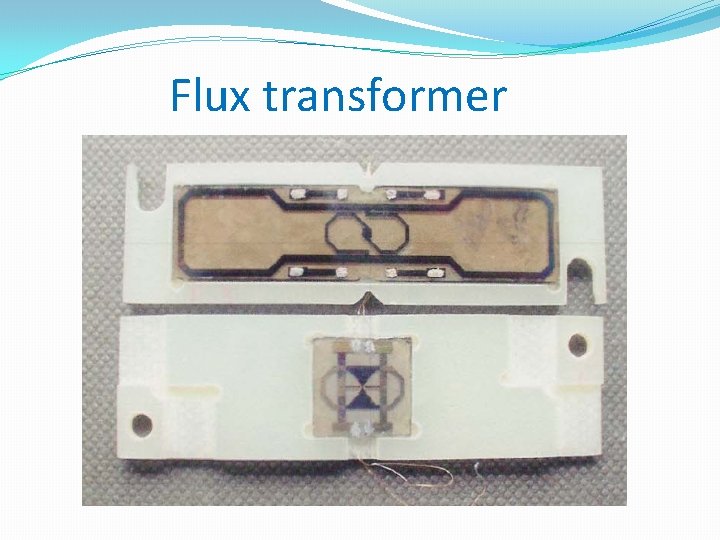
Flux transformer
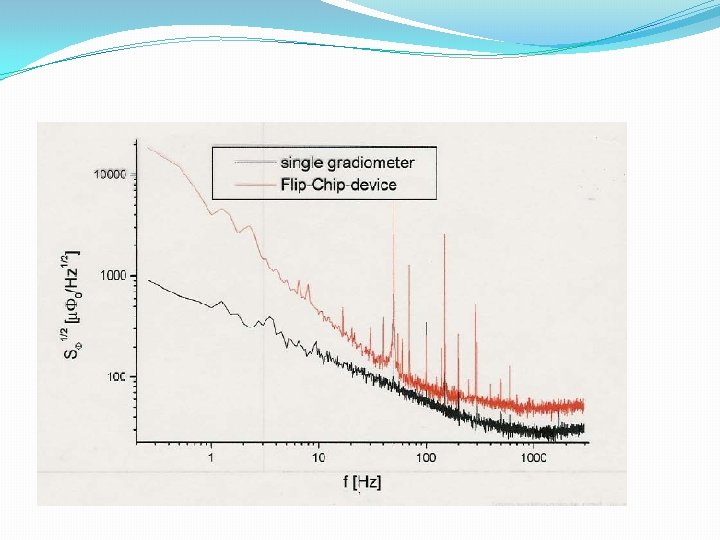
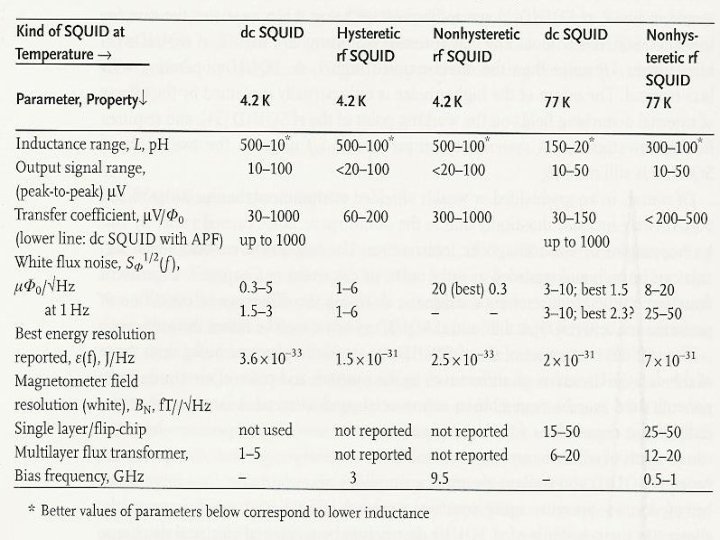
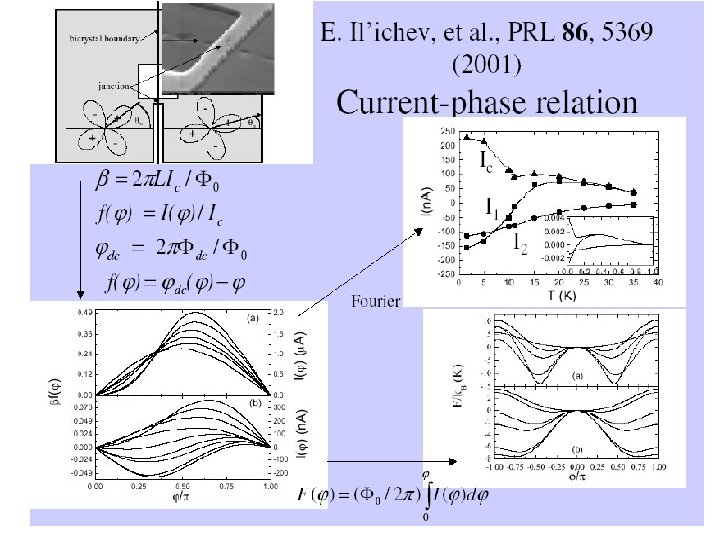
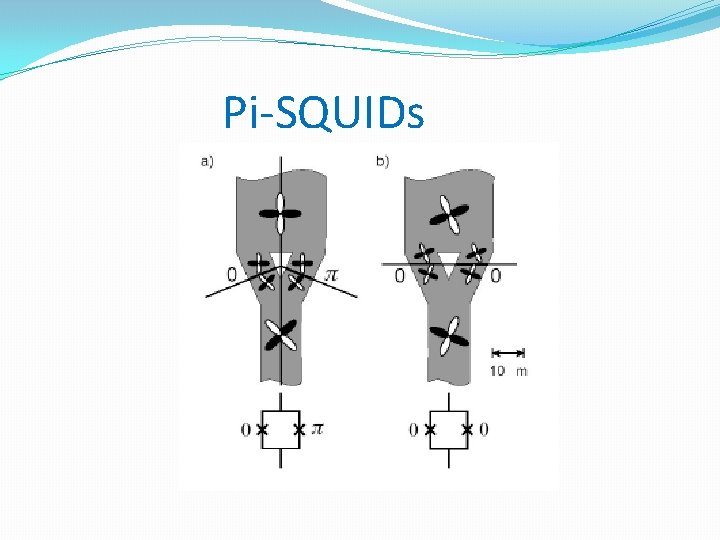
Pi-SQUIDs
![Chesca [Chesca]](https://slidetodoc.com/presentation_image_h2/81def57c997796372a709fb5fc171f16/image-68.jpg)
[Chesca]
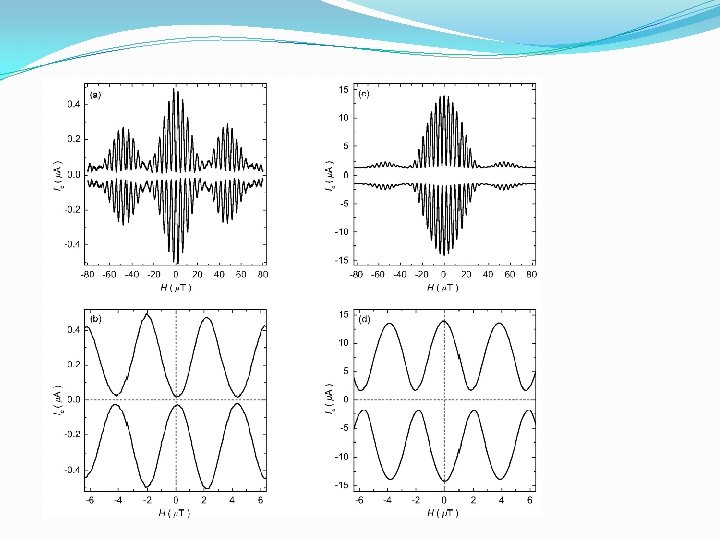
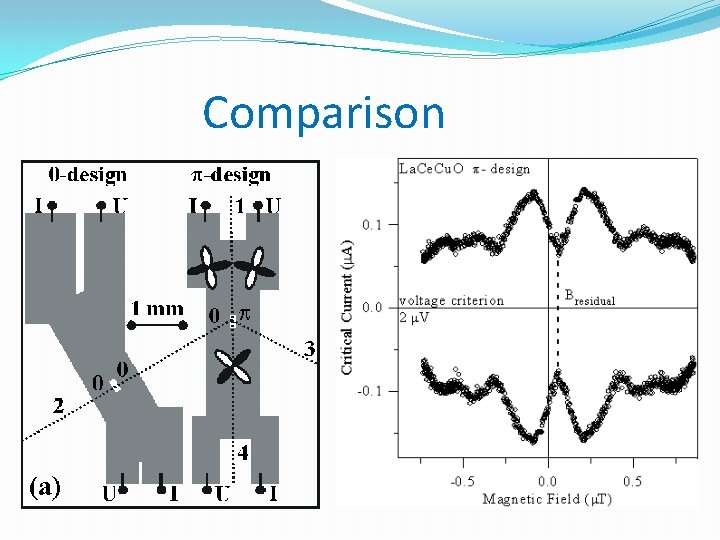
Comparison
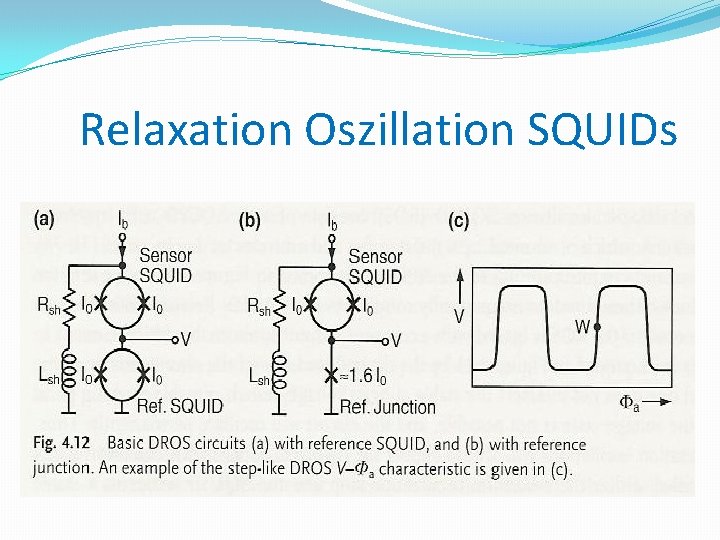
Relaxation Oszillation SQUIDs
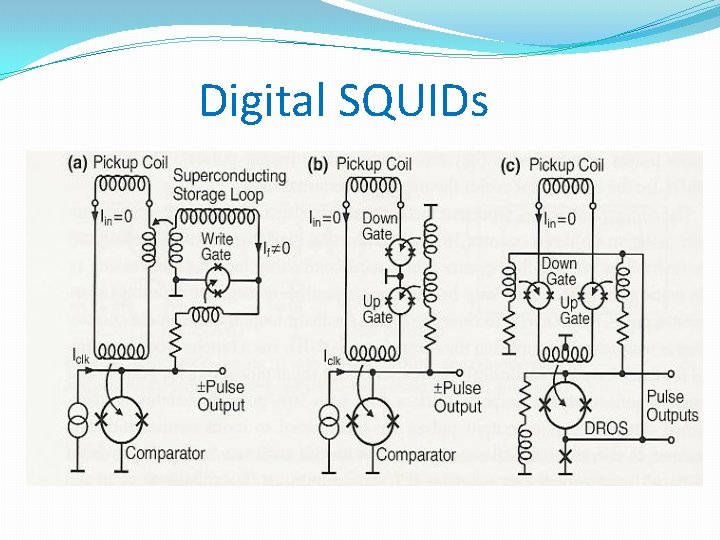
Digital SQUIDs
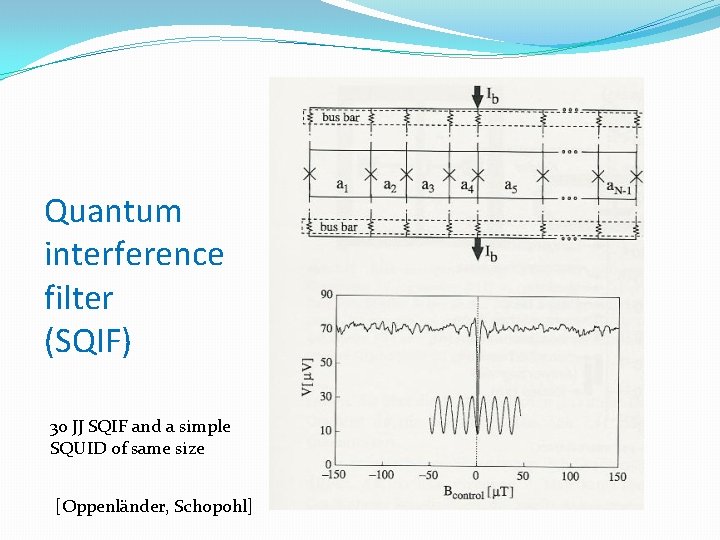
Quantum interference filter (SQIF) 30 JJ SQIF and a simple SQUID of same size [Oppenländer, Schopohl]
![Serial SQIF Schultze Serial SQIF [Schultze]](https://slidetodoc.com/presentation_image_h2/81def57c997796372a709fb5fc171f16/image-74.jpg)
Serial SQIF [Schultze]
![2 p T Hz IPHT Jena 2 p. T / √ Hz [IPHT Jena]}](https://slidetodoc.com/presentation_image_h2/81def57c997796372a709fb5fc171f16/image-75.jpg)
2 p. T / √ Hz [IPHT Jena]}
![2 D SQIF array R Fagaly 2 D- SQIF array [R. Fagaly]](https://slidetodoc.com/presentation_image_h2/81def57c997796372a709fb5fc171f16/image-76.jpg)
2 D- SQIF array [R. Fagaly]
![BiSQUID R Fagaly Bi-SQUID [R. Fagaly]](https://slidetodoc.com/presentation_image_h2/81def57c997796372a709fb5fc171f16/image-77.jpg)
Bi-SQUID [R. Fagaly]
Superconducting electronics
Superconducting devices in quantum optics
Superconducting tunnel junction
Superconducting energy gap
Superconducting tunnel junction
Ginzburg landau
Superconducting tunnel junction
Western superconducting
Oxford superconducting technology
Superconducting tunnel junction
Youtube.com
Rbbb vs lbbb
Josephson c700s
Josephson junction
Josephson junctions
Josephson devices
Josephson junction
Josephson effect
Högerställd elaxel
Alexander golubov
Josephson institute of ethics
Josephson junction
Michael josephson ethics
Marvin josephson
Quantum physics vs quantum mechanics
Quantum physics vs quantum mechanics
Quantum computing ut austin
Open problems in quantum computing
Quantum computing
Gil kalai quantum computing
Tsp
Hartmut klauck
Mit quantum computing
Dilbert quantum computing
Quantum computing progress and prospects
Quantum computing and the entanglement frontier
Online learning of quantum states
Mit quantum computing
Haskell quantum computing
Michael nielsen quantum
Quantum computing prerequisites
Cern openlab quantum computing
Quantum computing meaning
Gil kalai quantum computing
Conventional computing and intelligent computing
Mgi electronics
1 source electronics
Where was ben abbott born
Arithmetic building blocks
Power electronics
Electronics 2
Binary number system in digital electronics
Giuliana rizzo
Semiconductor memories in digital electronics
Analog electronics
Bcm electronics
De morgan
Analog electronics
Setup time and hold time in digital electronics
Non weighted codes examples
Institute
Power transfer system
Electronics materials solutions
Reimax electronics
Pixel electronics
Frc electronics diagram
3m acf
Petsys electronics
Department of electronics & information technology
Rydertrac
Advanced automotive electric
Graduate institute of electronics engineering
Derivation of continuity equation in semiconductor
Introduction to power electronics
Ac voltage controller and cycloconverter
Boolen algebra
State machines digital electronics
Gunther electronics