Quantum Computing Scott Aaronson UT Austin Lakeway Mens
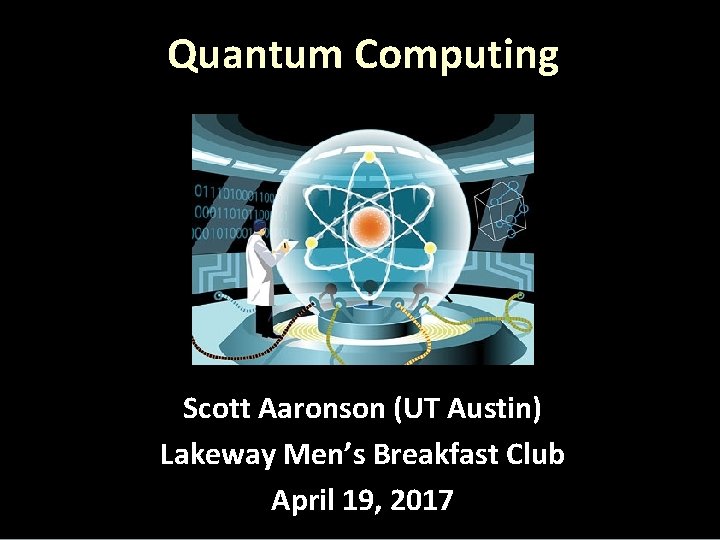
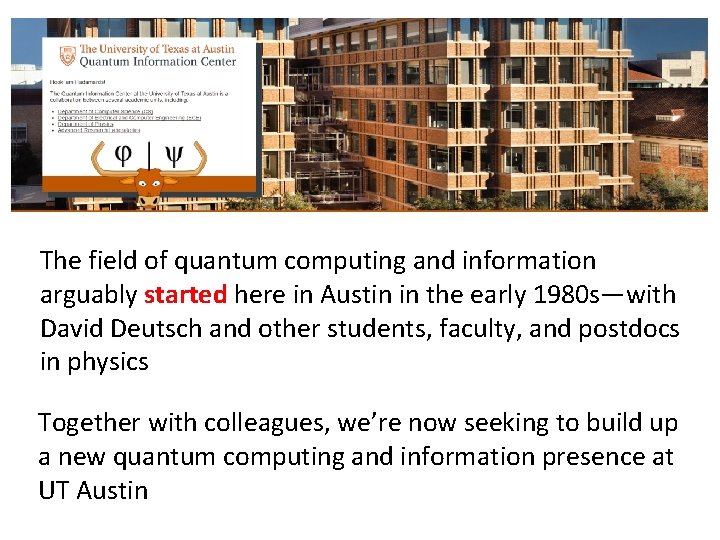
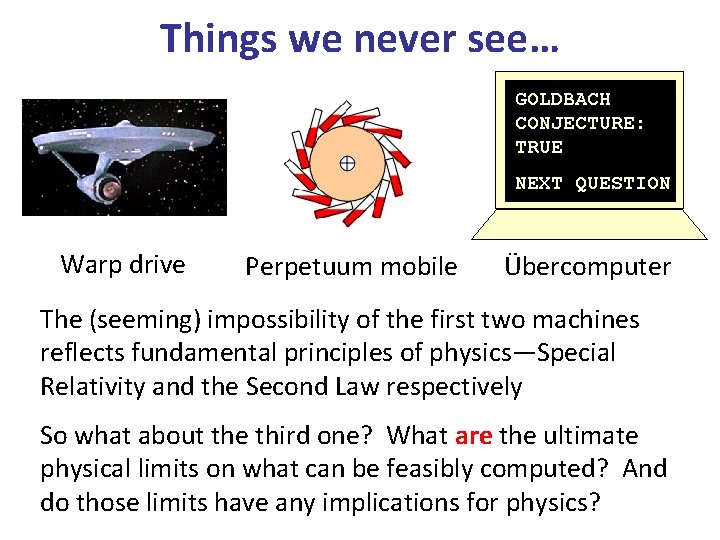
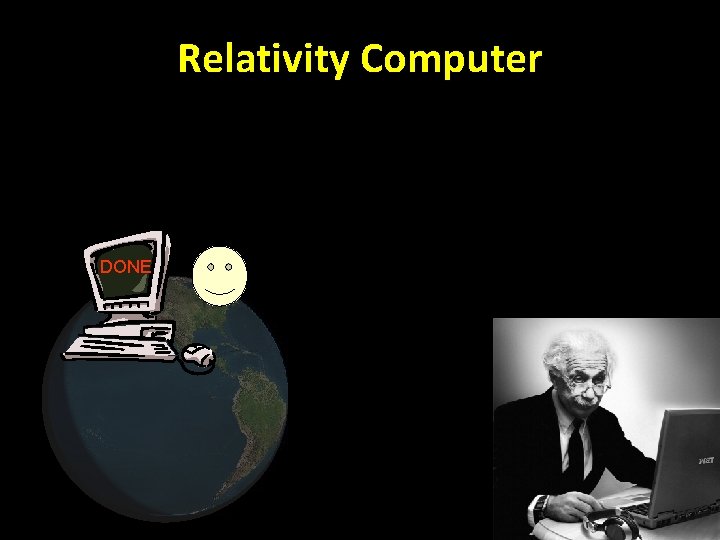
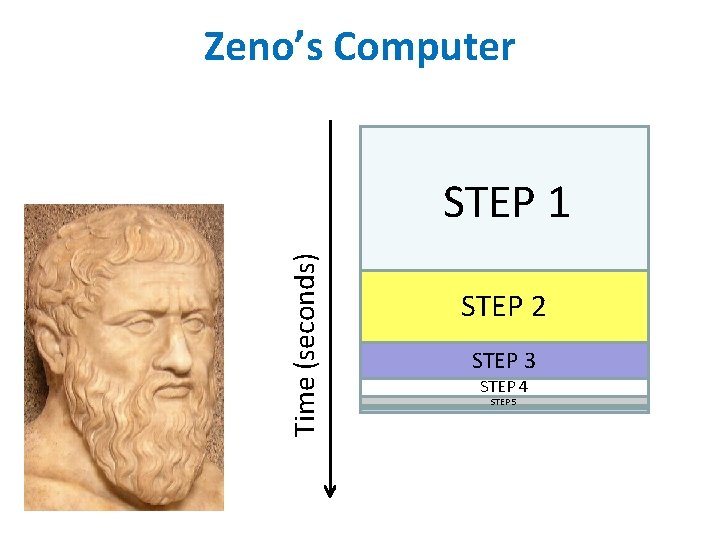
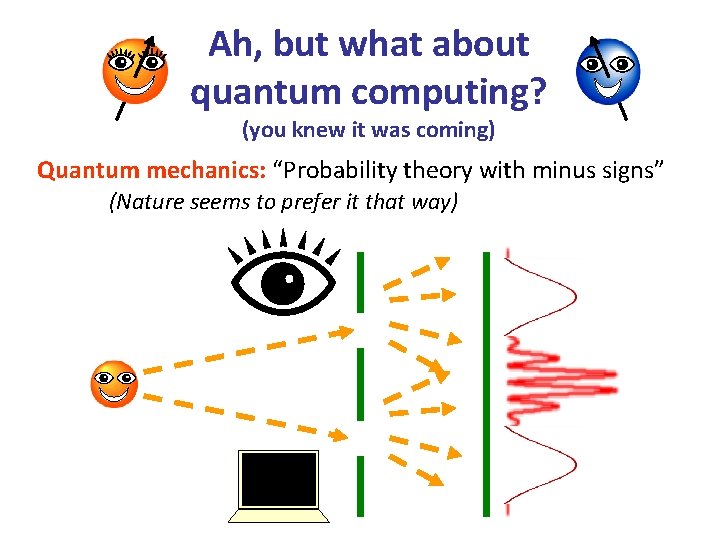
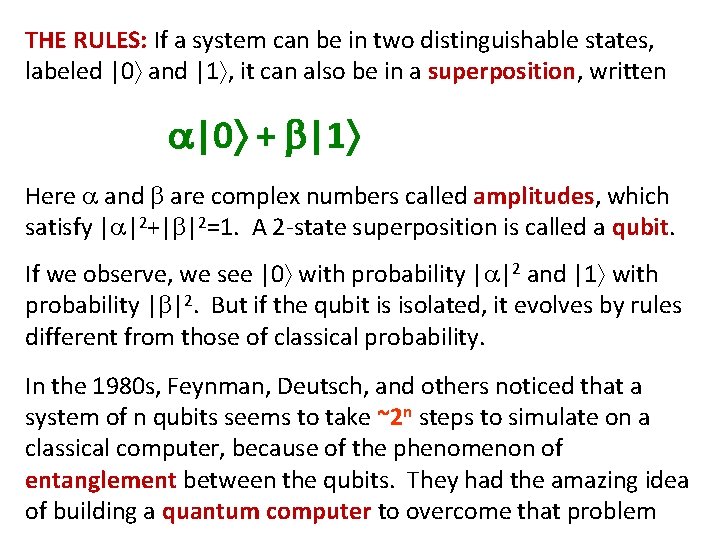
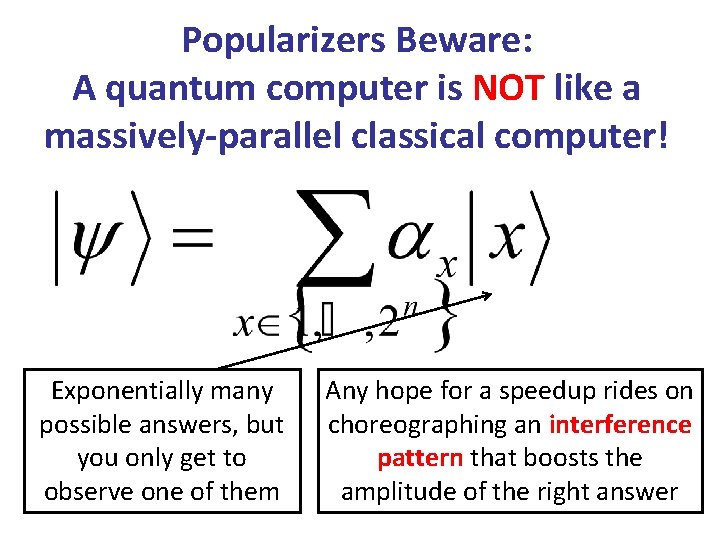
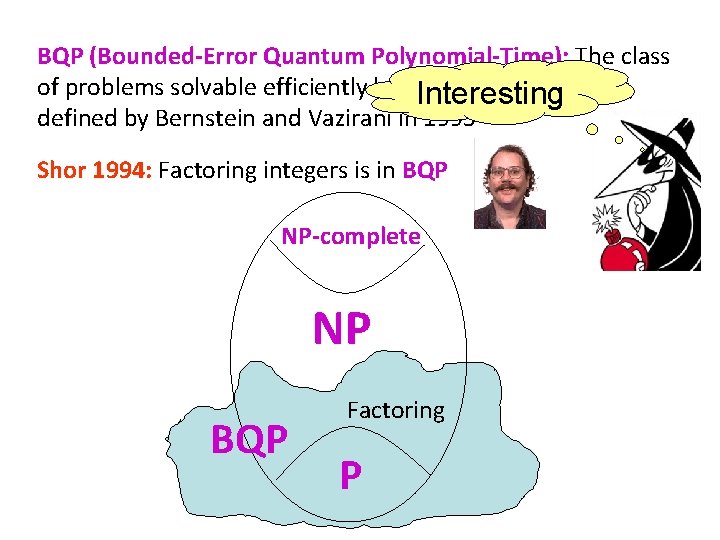
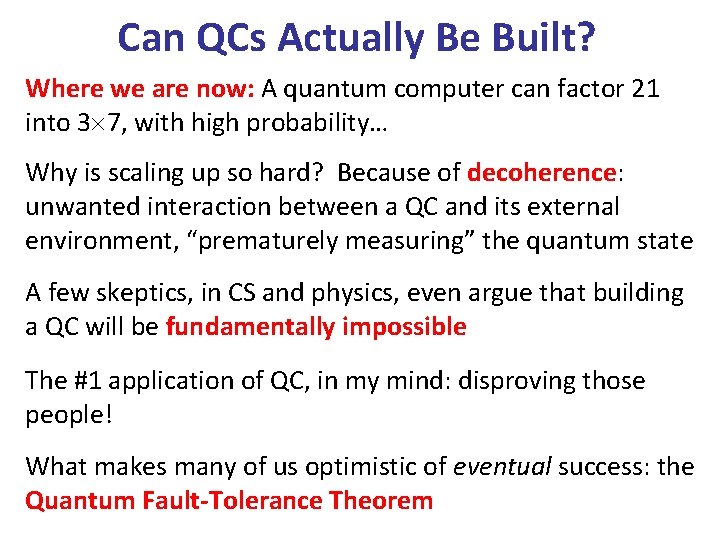
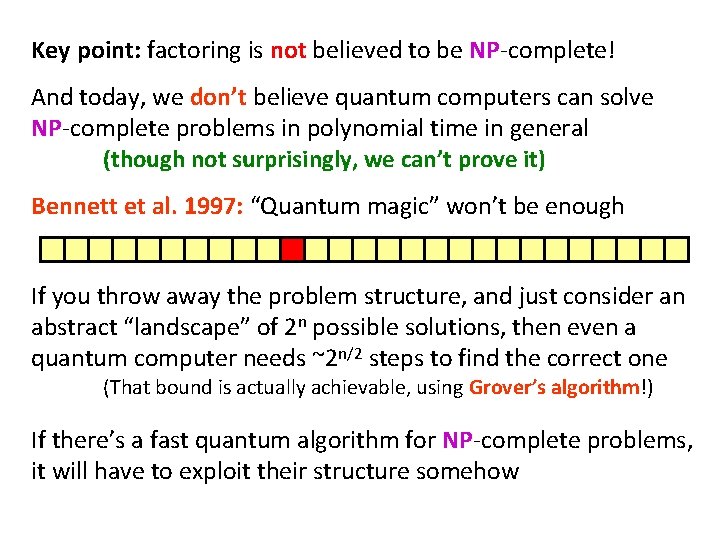
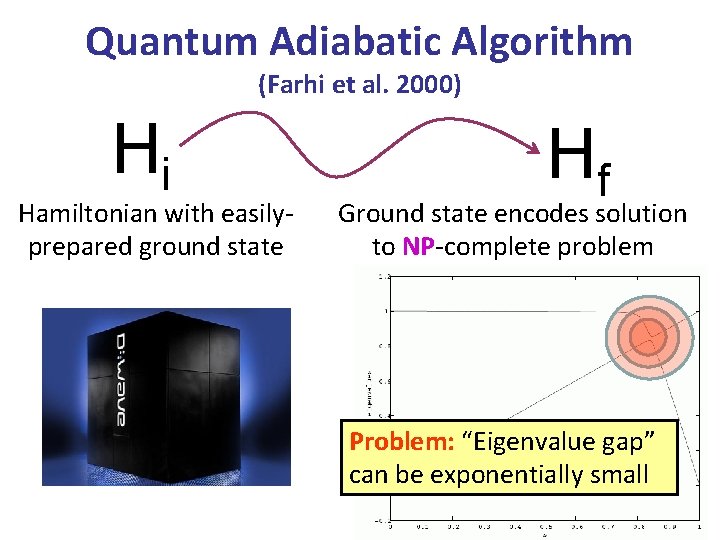
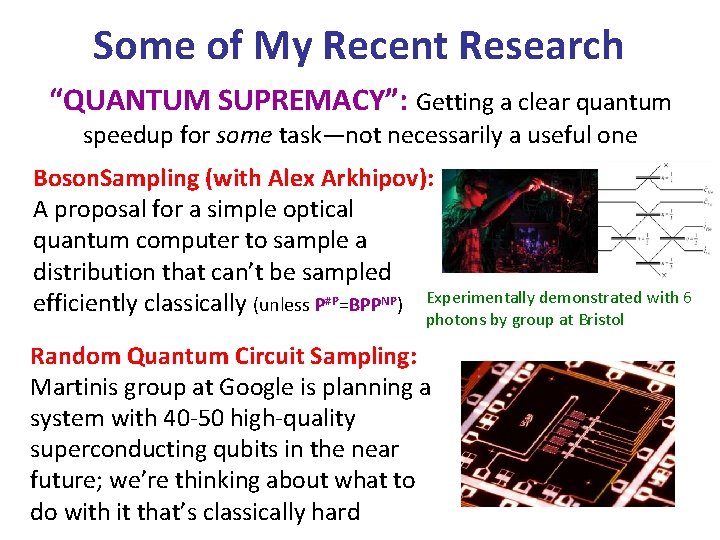
- Slides: 13
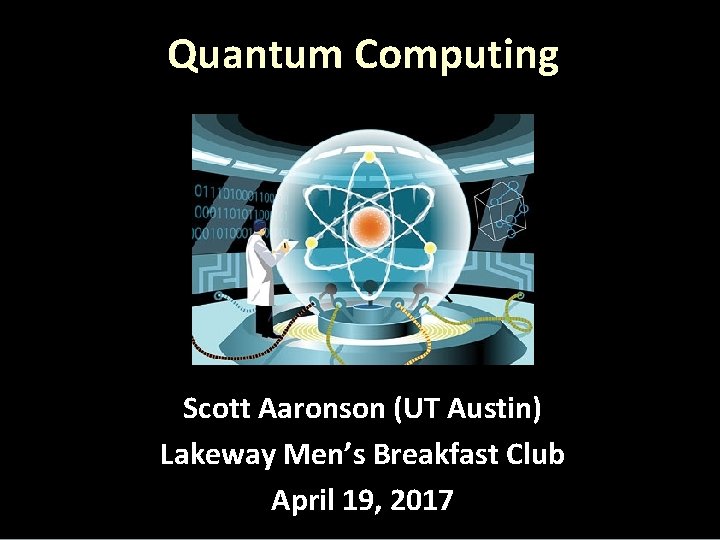
Quantum Computing Scott Aaronson (UT Austin) Lakeway Men’s Breakfast Club April 19, 2017
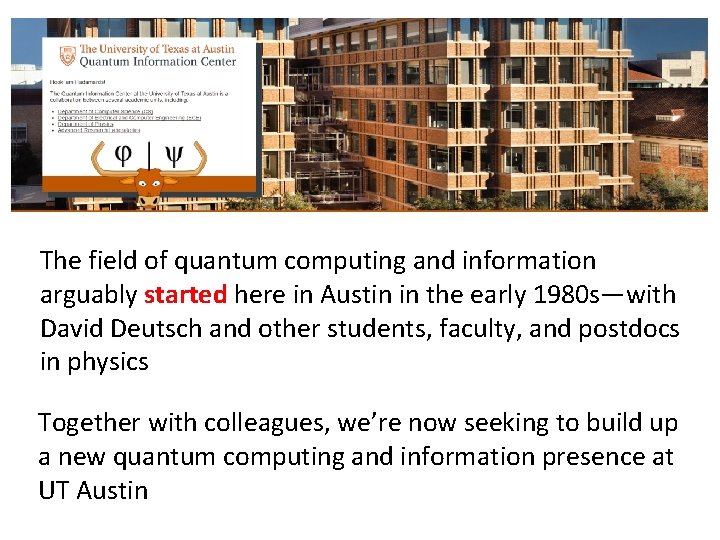
The field of quantum computing and information arguably started here in Austin in the early 1980 s—with David Deutsch and other students, faculty, and postdocs in physics Together with colleagues, we’re now seeking to build up a new quantum computing and information presence at UT Austin
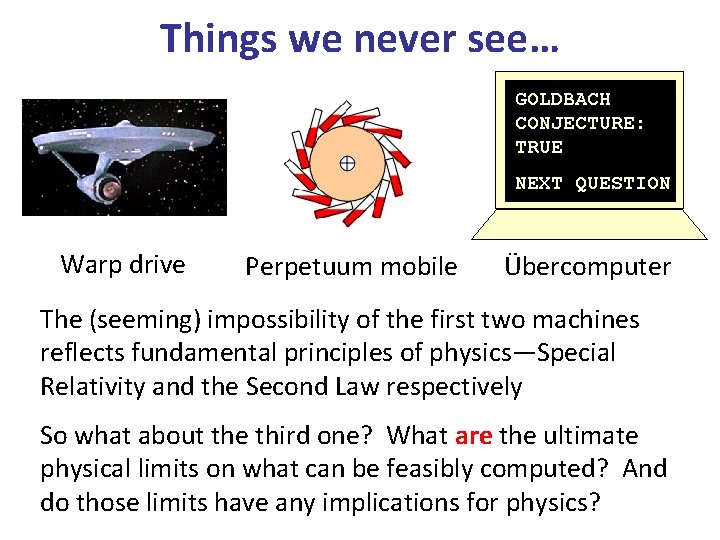
Things we never see… GOLDBACH CONJECTURE: TRUE NEXT QUESTION Warp drive Perpetuum mobile Übercomputer The (seeming) impossibility of the first two machines reflects fundamental principles of physics—Special Relativity and the Second Law respectively So what about the third one? What are the ultimate physical limits on what can be feasibly computed? And do those limits have any implications for physics?
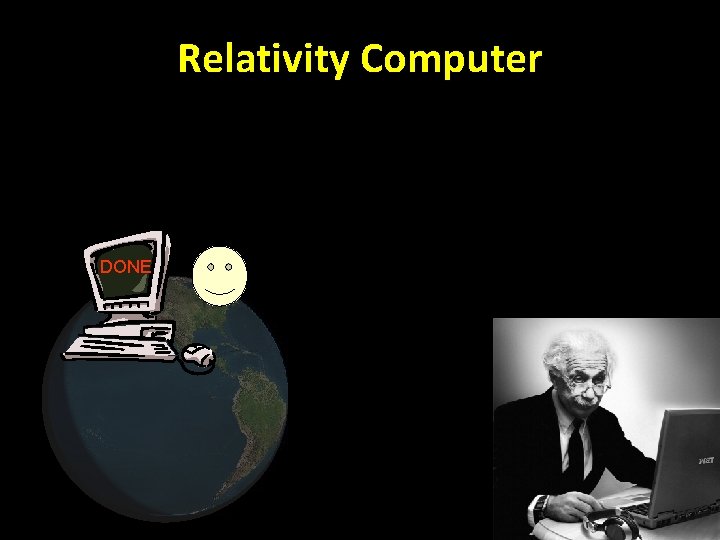
Relativity Computer DONE
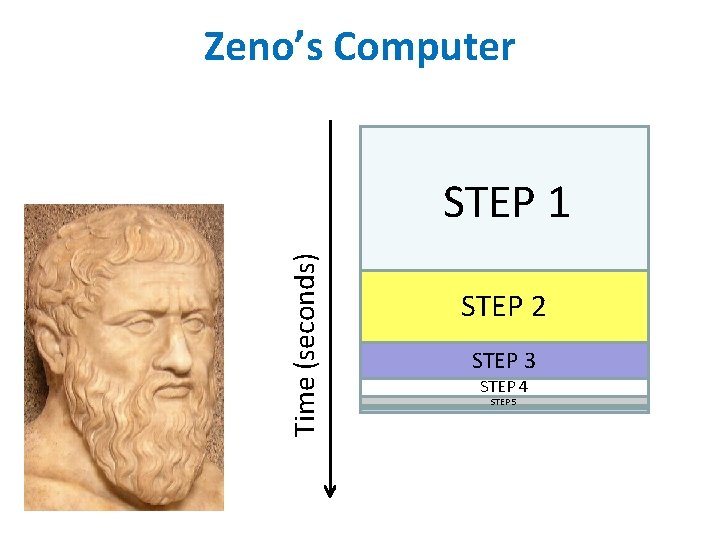
Zeno’s Computer Time (seconds) STEP 1 STEP 2 STEP 3 STEP 4 STEP 5
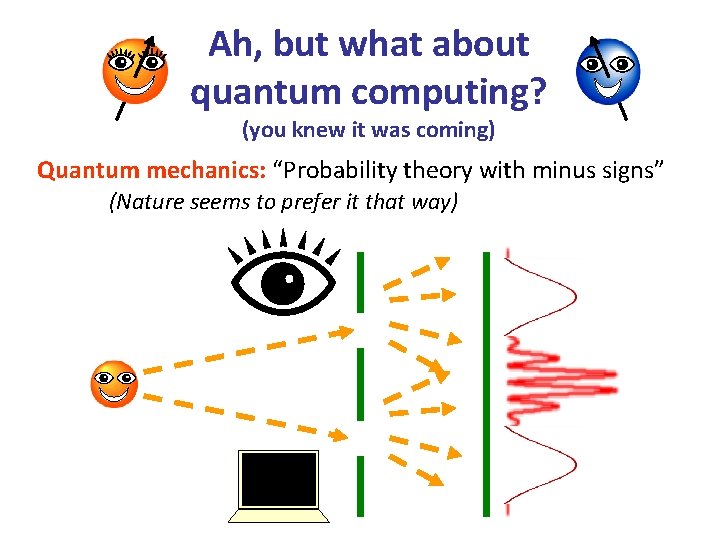
Ah, but what about quantum computing? (you knew it was coming) Quantum mechanics: “Probability theory with minus signs” (Nature seems to prefer it that way)
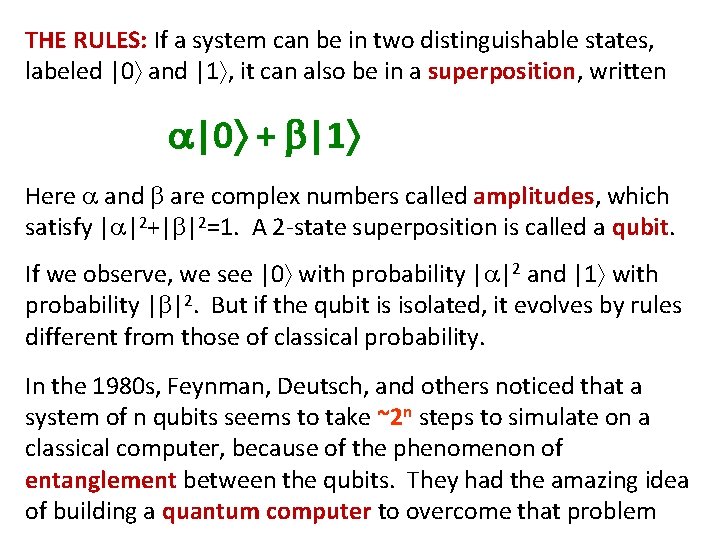
THE RULES: If a system can be in two distinguishable states, labeled |0 and |1 , it can also be in a superposition, written |0 + |1 Here and are complex numbers called amplitudes, which satisfy | |2+| |2=1. A 2 -state superposition is called a qubit. If we observe, we see |0 with probability | |2 and |1 with probability | |2. But if the qubit is isolated, it evolves by rules different from those of classical probability. In the 1980 s, Feynman, Deutsch, and others noticed that a system of n qubits seems to take ~2 n steps to simulate on a classical computer, because of the phenomenon of entanglement between the qubits. They had the amazing idea of building a quantum computer to overcome that problem
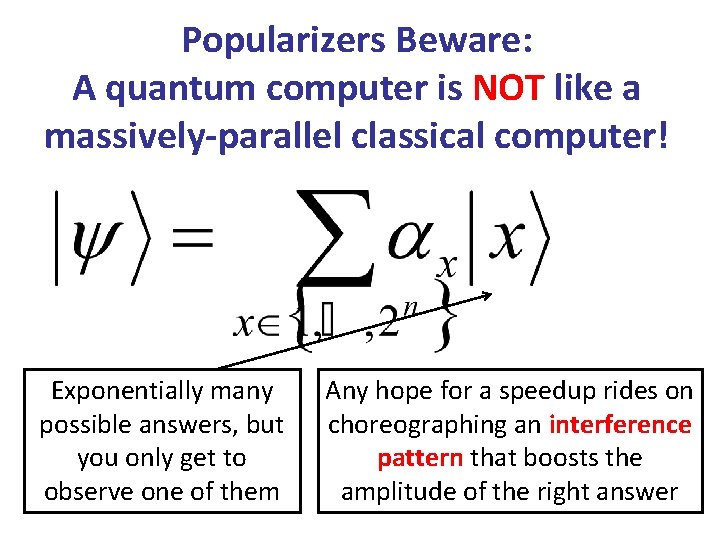
Popularizers Beware: A quantum computer is NOT like a massively-parallel classical computer! Exponentially many possible answers, but you only get to observe one of them Any hope for a speedup rides on choreographing an interference pattern that boosts the amplitude of the right answer
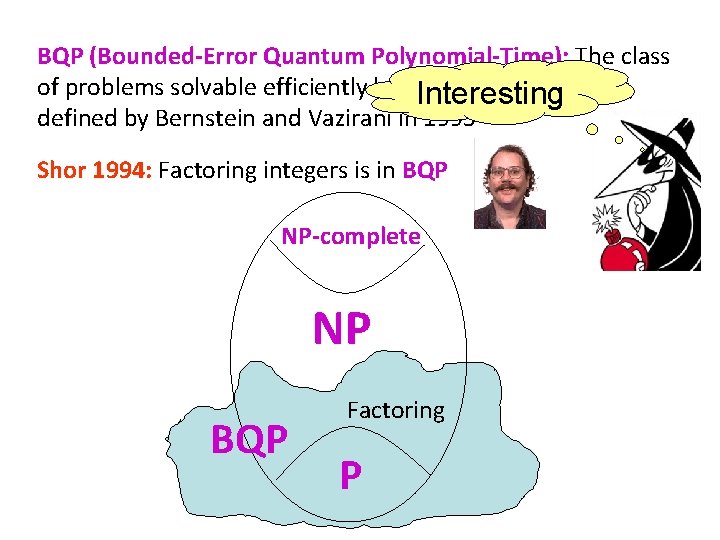
BQP (Bounded-Error Quantum Polynomial-Time): The class of problems solvable efficiently by a. Interesting quantum computer, defined by Bernstein and Vazirani in 1993 Shor 1994: Factoring integers is in BQP NP-complete NP BQP Factoring P
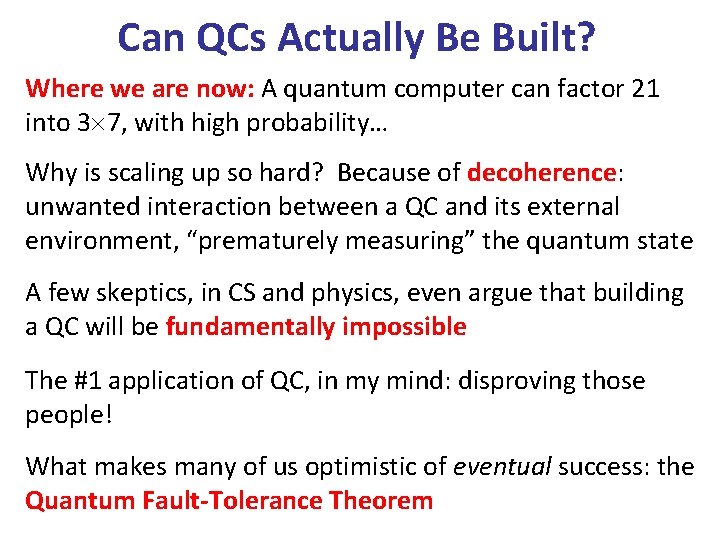
Can QCs Actually Be Built? Where we are now: A quantum computer can factor 21 into 3 7, with high probability… Why is scaling up so hard? Because of decoherence: unwanted interaction between a QC and its external environment, “prematurely measuring” the quantum state A few skeptics, in CS and physics, even argue that building a QC will be fundamentally impossible The #1 application of QC, in my mind: disproving those people! What makes many of us optimistic of eventual success: the Quantum Fault-Tolerance Theorem
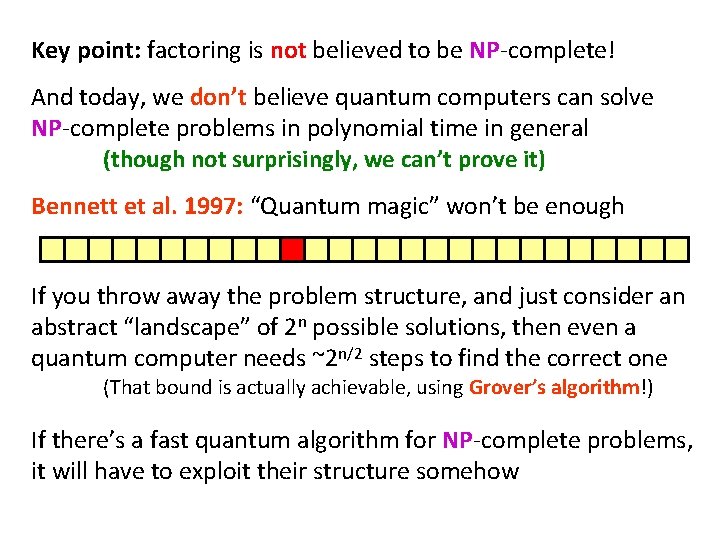
Key point: factoring is not believed to be NP-complete! And today, we don’t believe quantum computers can solve NP-complete problems in polynomial time in general (though not surprisingly, we can’t prove it) Bennett et al. 1997: “Quantum magic” won’t be enough If you throw away the problem structure, and just consider an abstract “landscape” of 2 n possible solutions, then even a quantum computer needs ~2 n/2 steps to find the correct one (That bound is actually achievable, using Grover’s algorithm!) If there’s a fast quantum algorithm for NP-complete problems, it will have to exploit their structure somehow
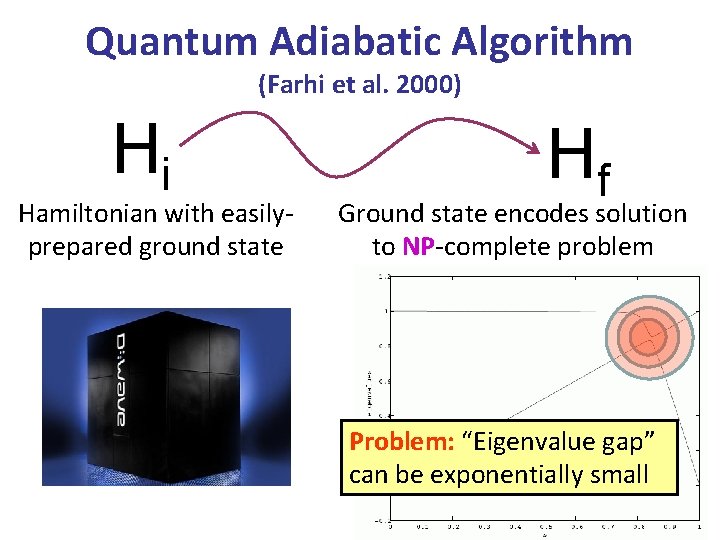
Quantum Adiabatic Algorithm (Farhi et al. 2000) Hi Hamiltonian with easilyprepared ground state Hf Ground state encodes solution to NP-complete problem Problem: “Eigenvalue gap” can be exponentially small
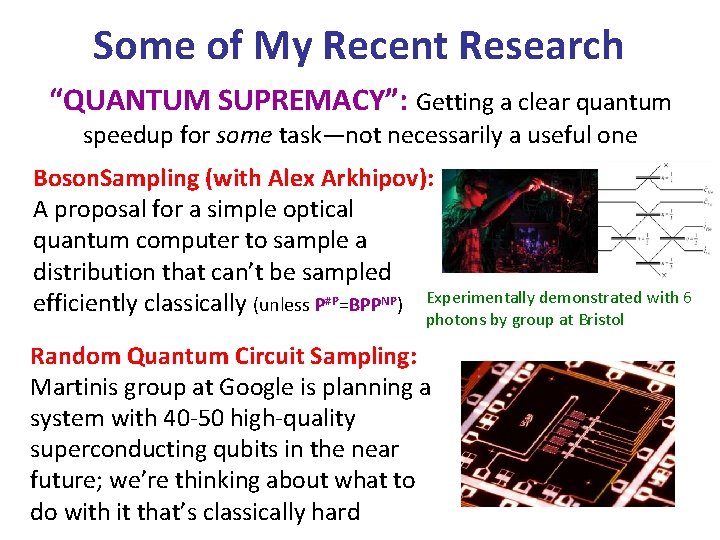
Some of My Recent Research “QUANTUM SUPREMACY”: Getting a clear quantum speedup for some task—not necessarily a useful one Boson. Sampling (with Alex Arkhipov): A proposal for a simple optical quantum computer to sample a distribution that can’t be sampled efficiently classically (unless P#P=BPPNP) Experimentally demonstrated with 6 photons by group at Bristol Random Quantum Circuit Sampling: Martinis group at Google is planning a system with 40 -50 high-quality superconducting qubits in the near future; we’re thinking about what to do with it that’s classically hard
Scott aaronson mit
Quantum computing ut austin
Ut austin quantum computing
Ut austin quantum computing
Np
"plf"
Lauren aaronson
Boson sampling aaronson
Boson sampling aaronson
Quantum physics vs quantum mechanics
Quantum physics vs quantum mechanics
Quantum computing progress and prospects
Cern openlab quantum computing
Open problems in quantum computing