Quantum computing and the entanglement frontier John Preskill
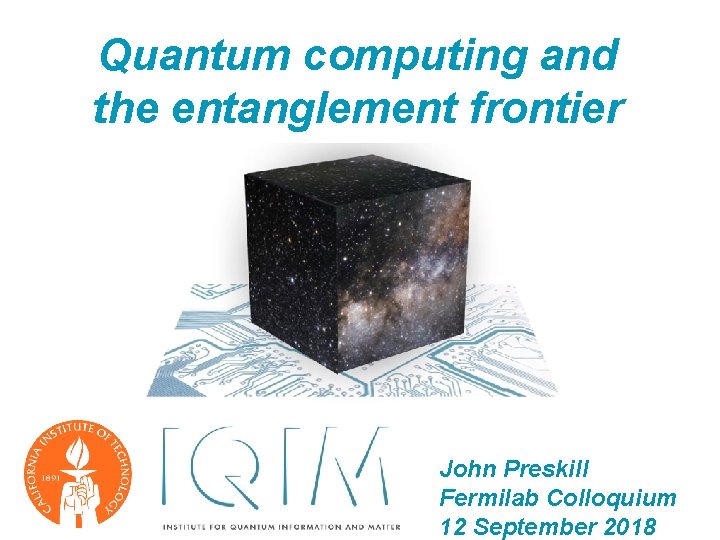
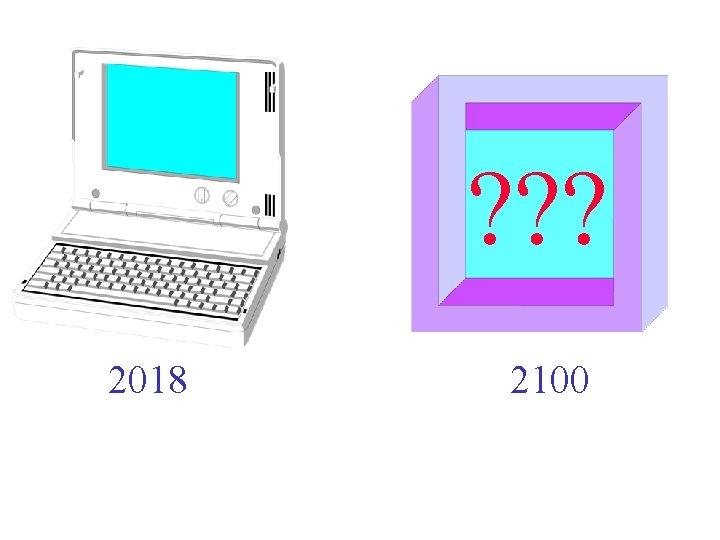
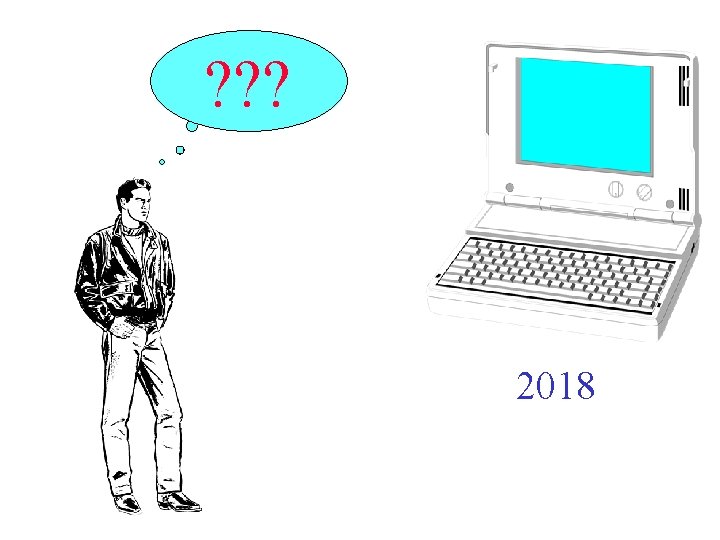
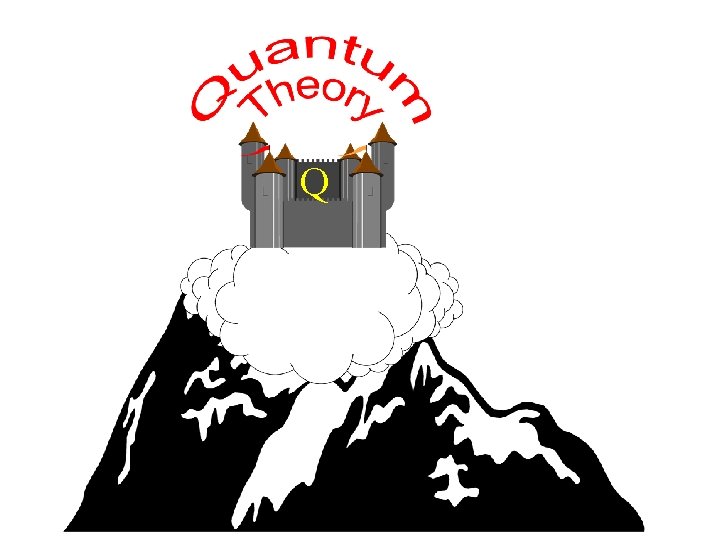
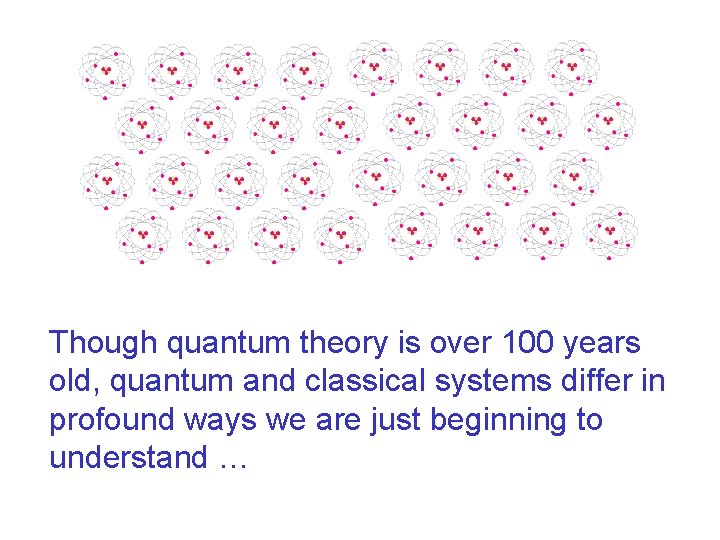
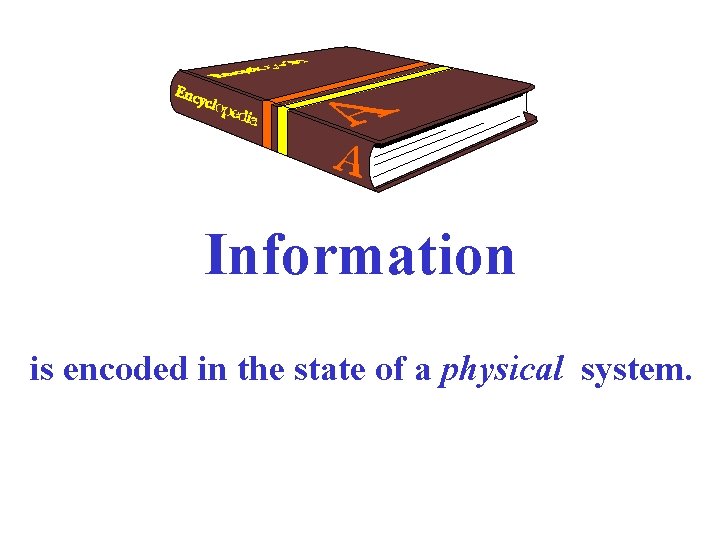
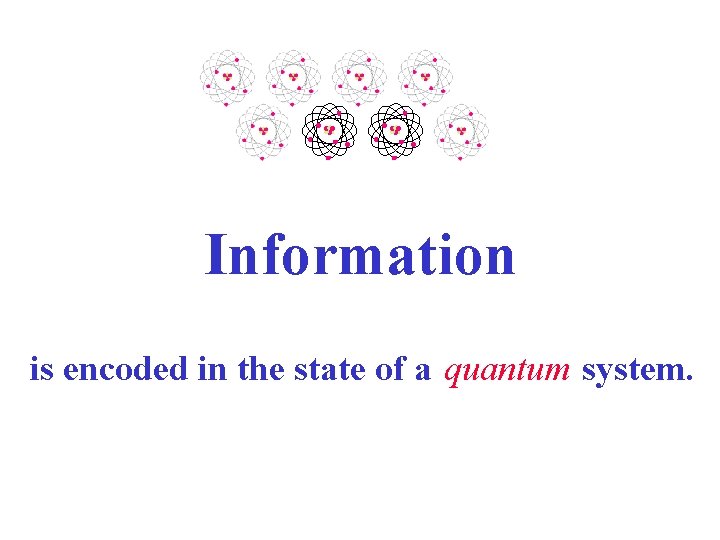
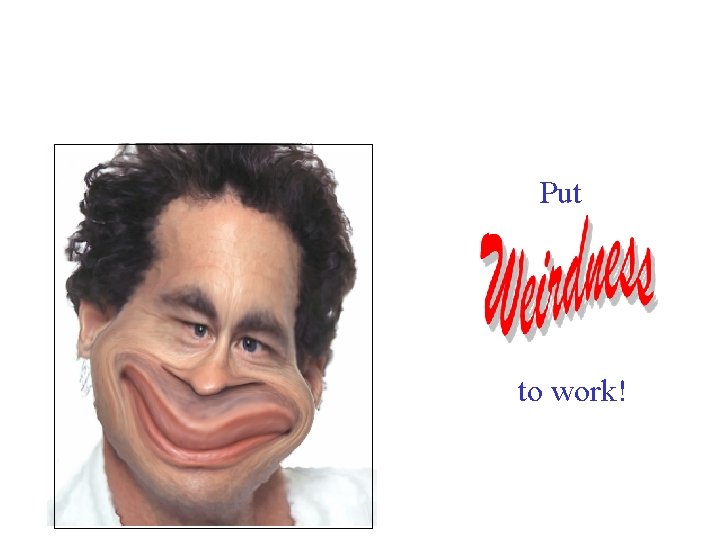
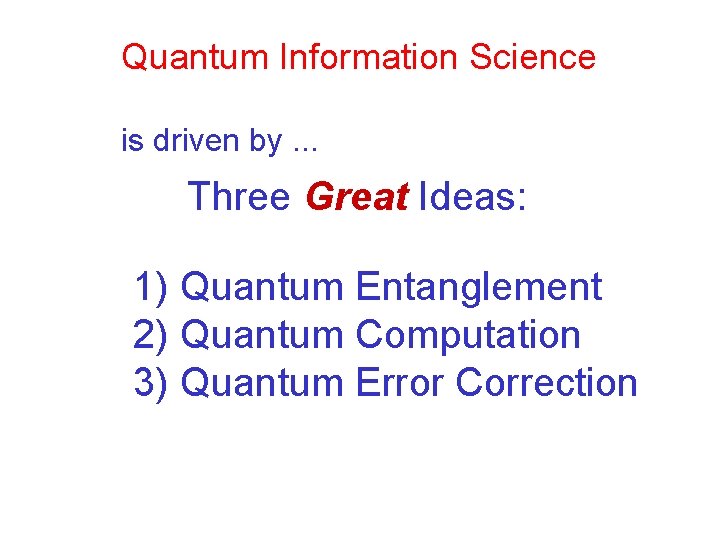
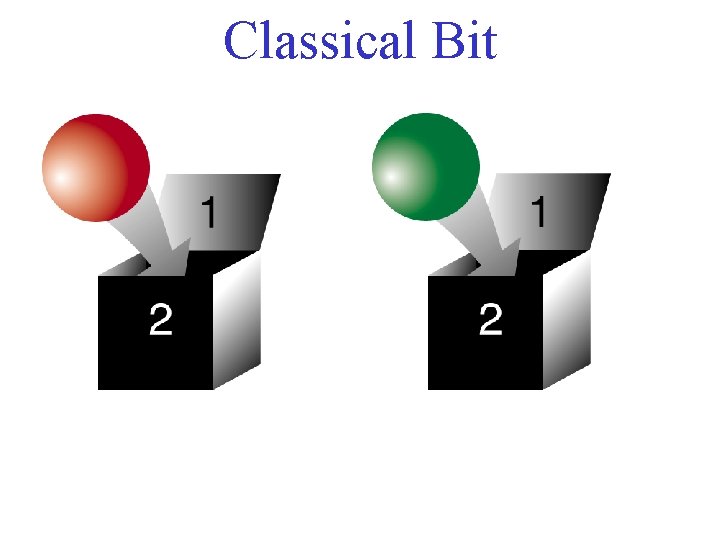
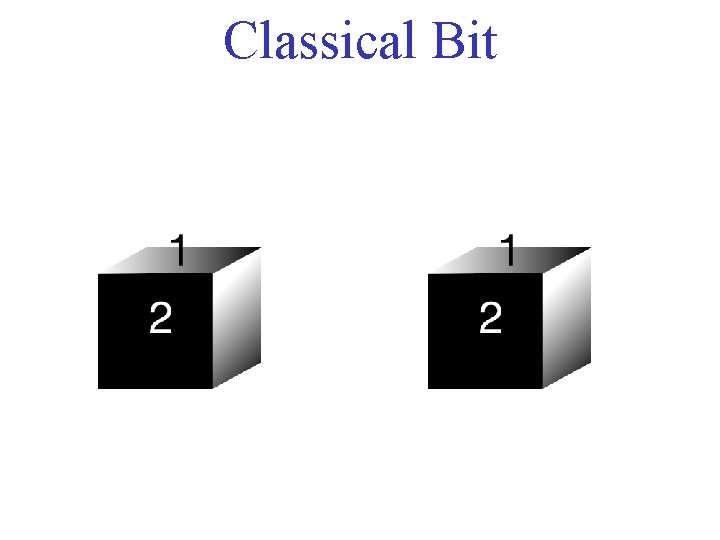
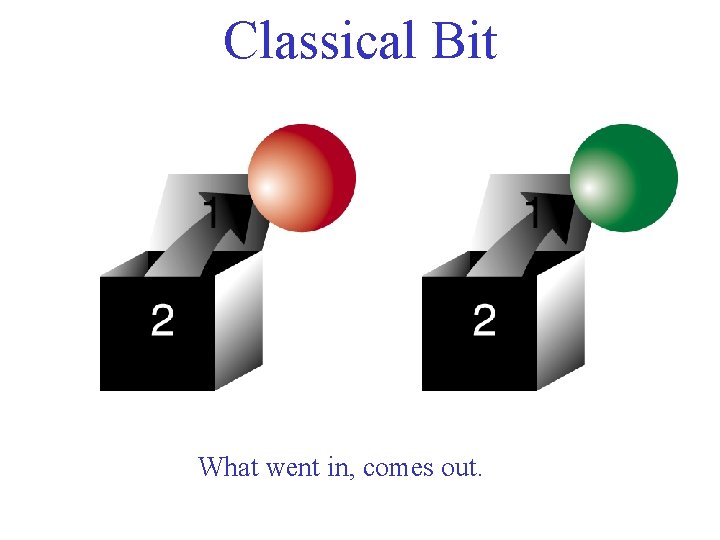
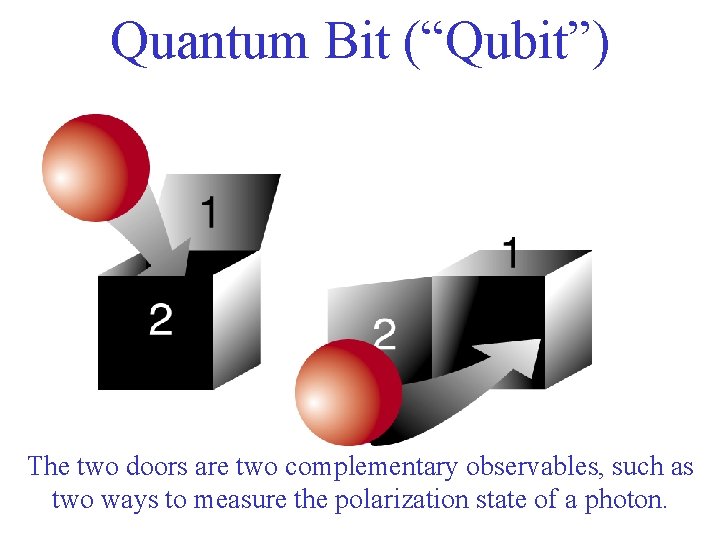
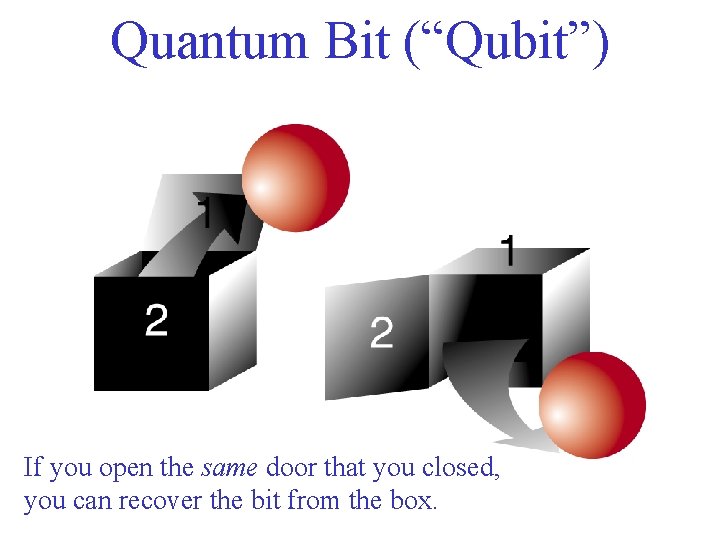
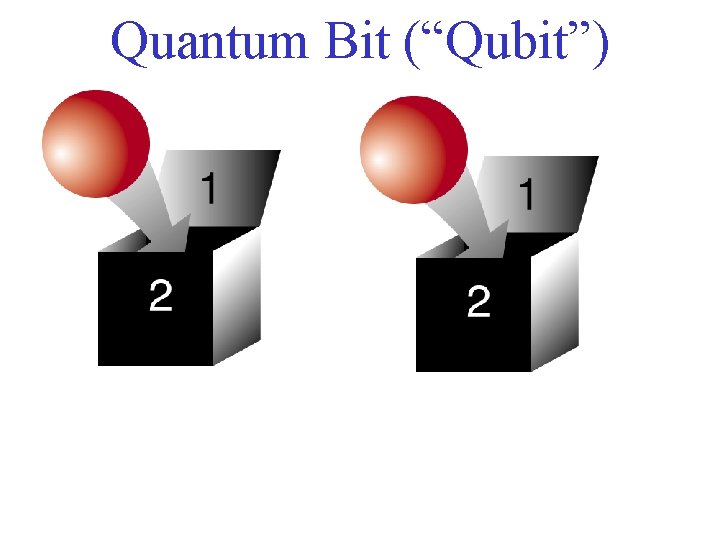
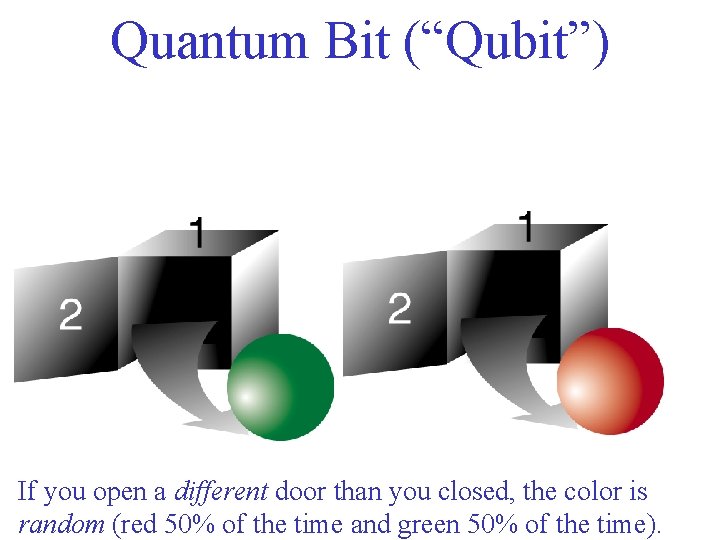
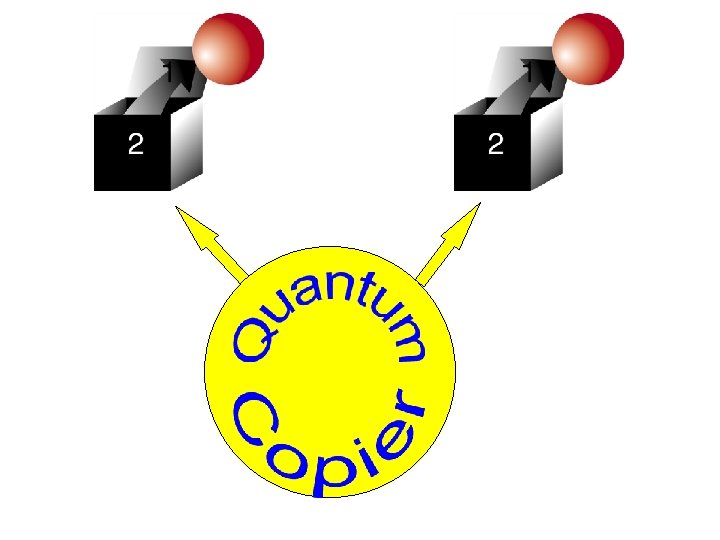
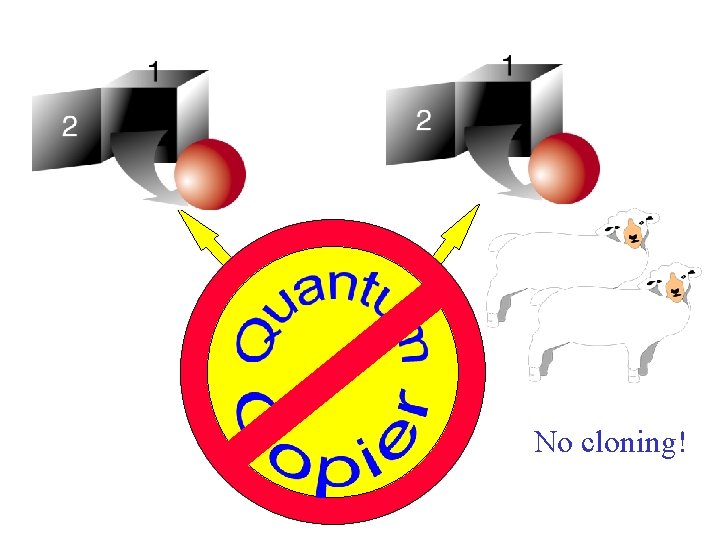
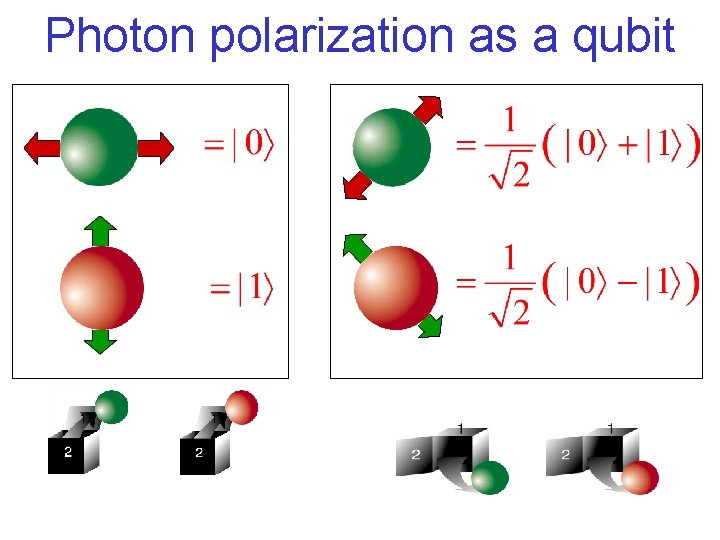
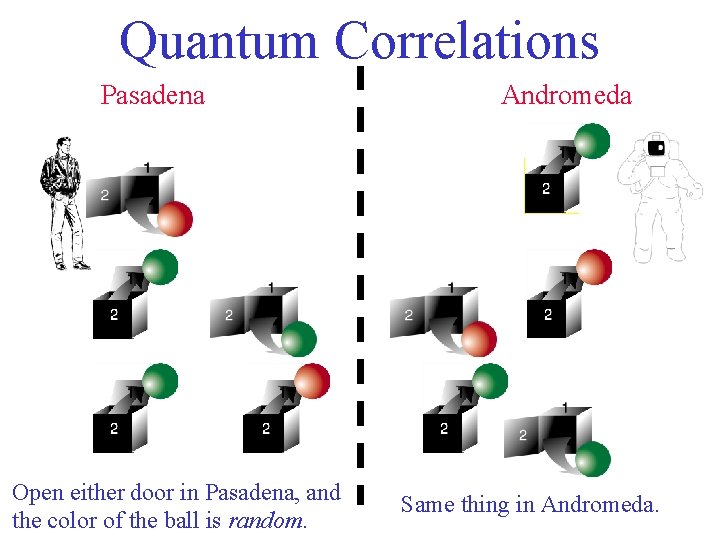
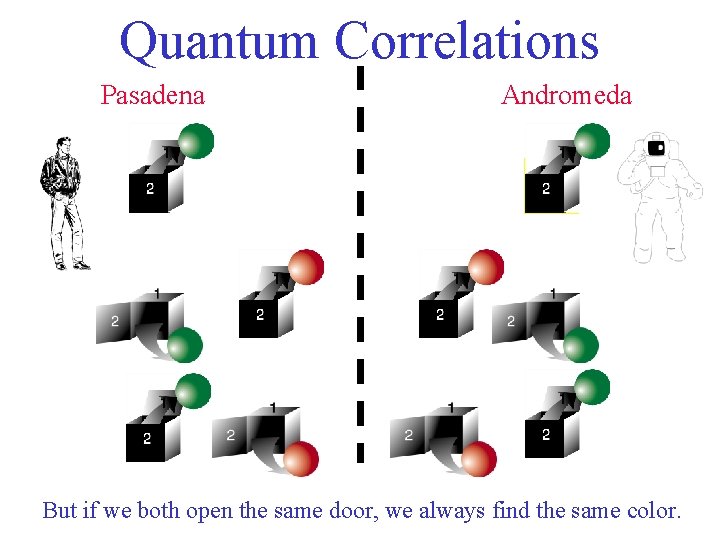
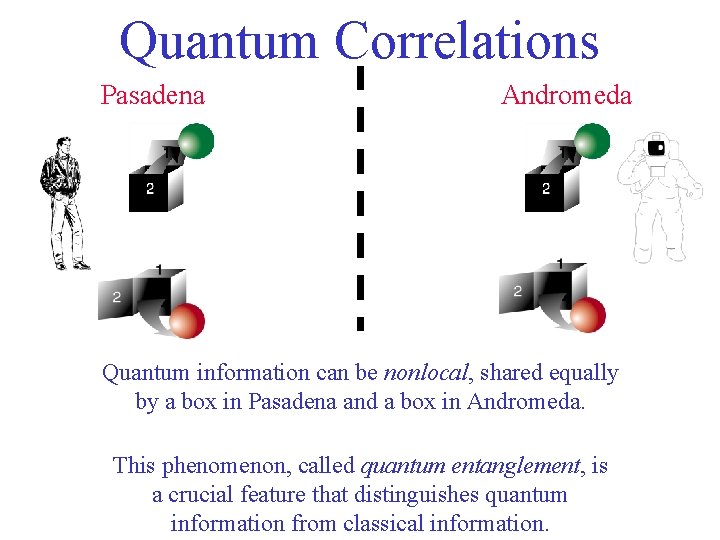
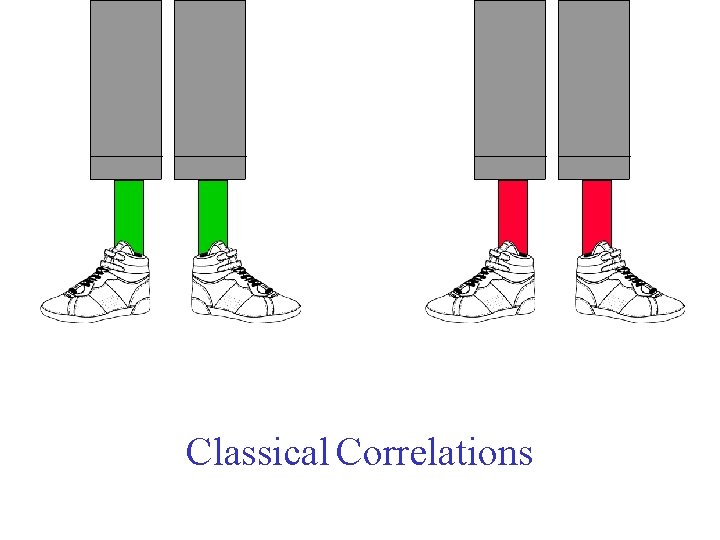
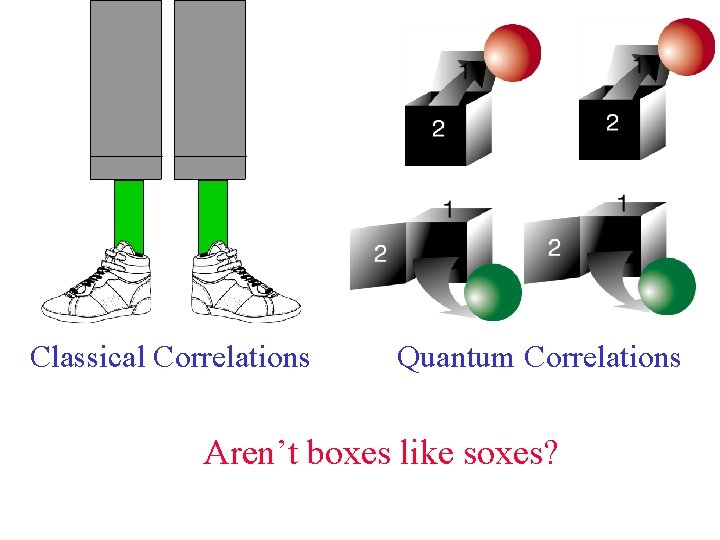
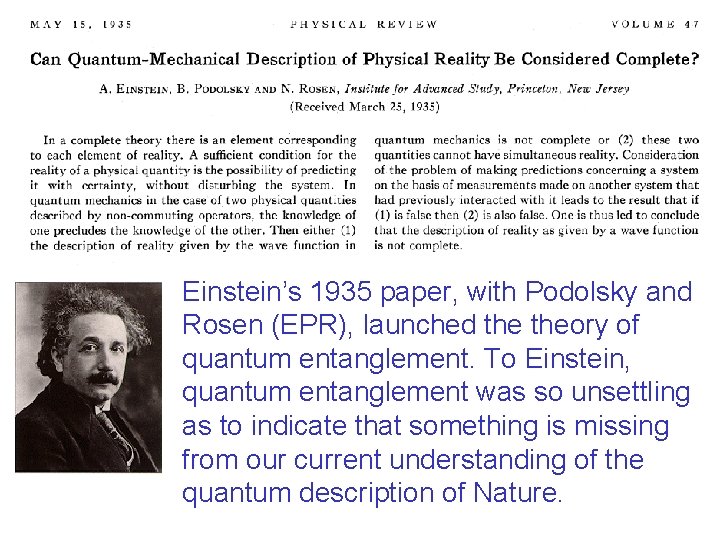
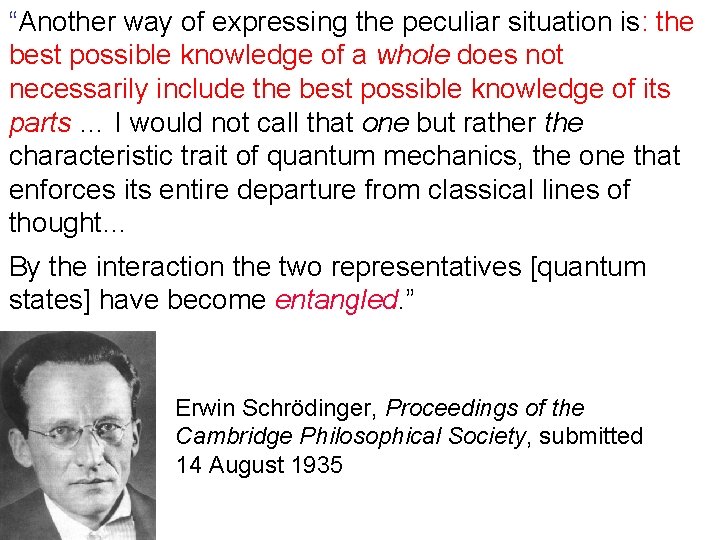
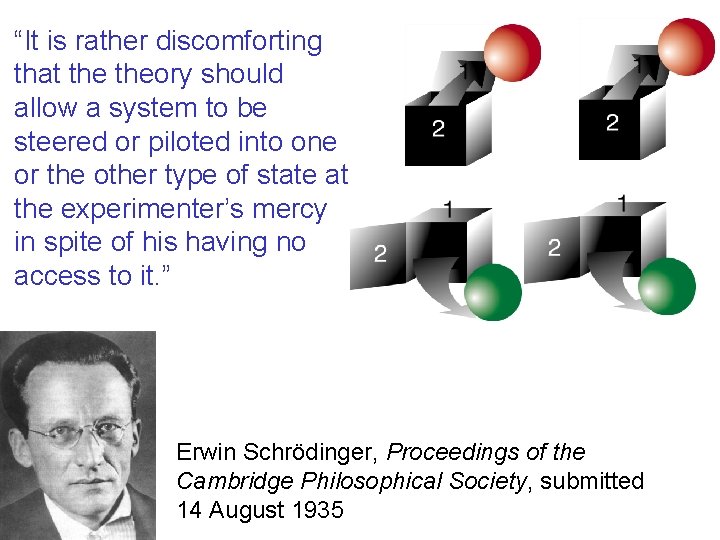
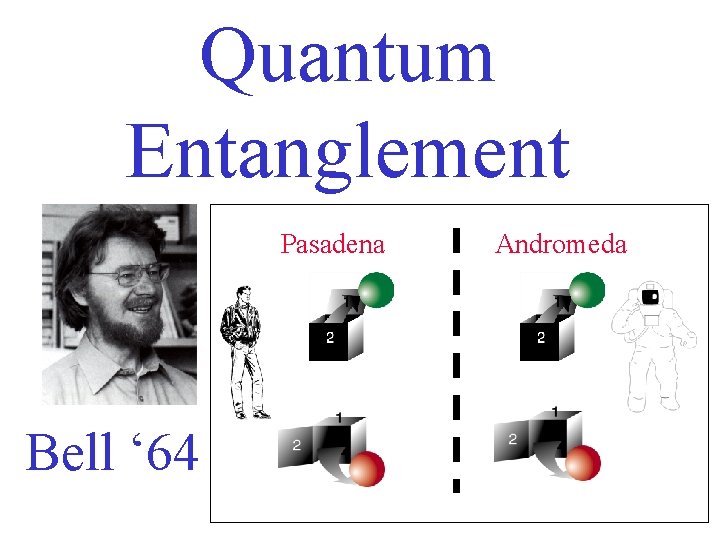
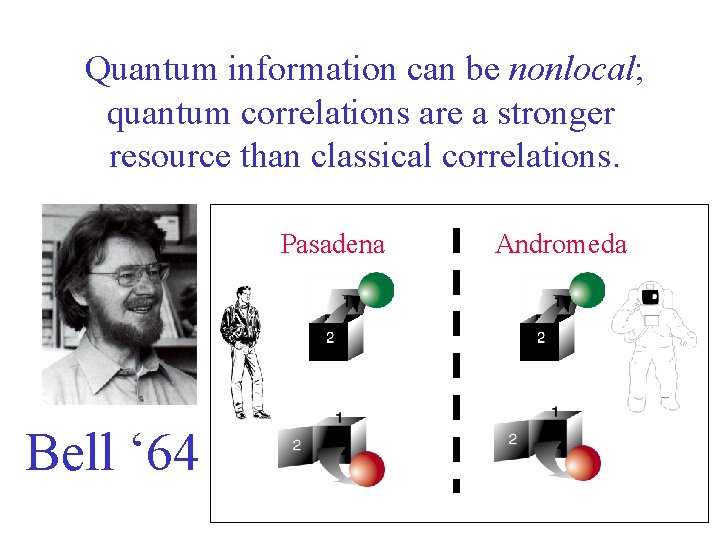
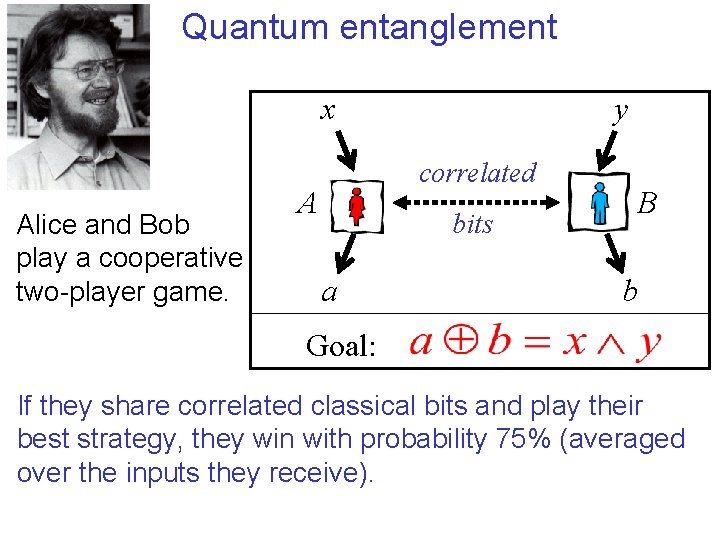
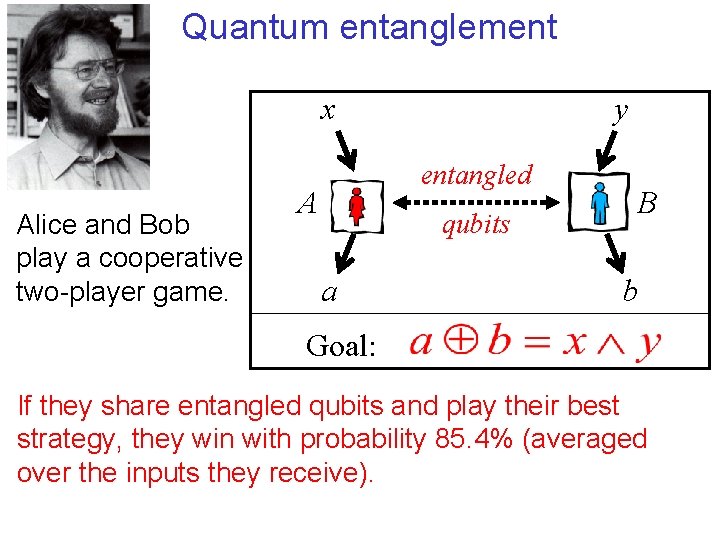
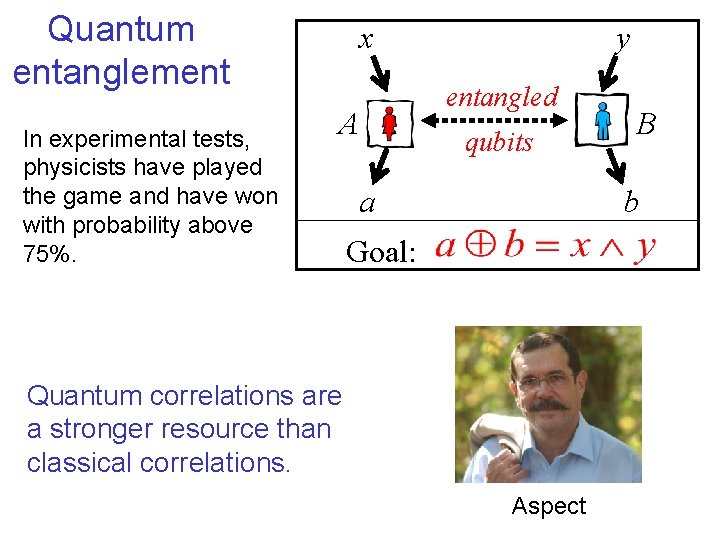
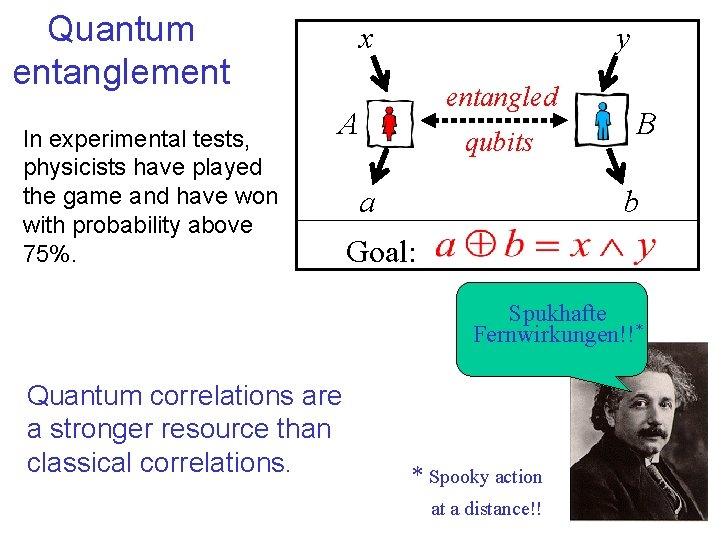
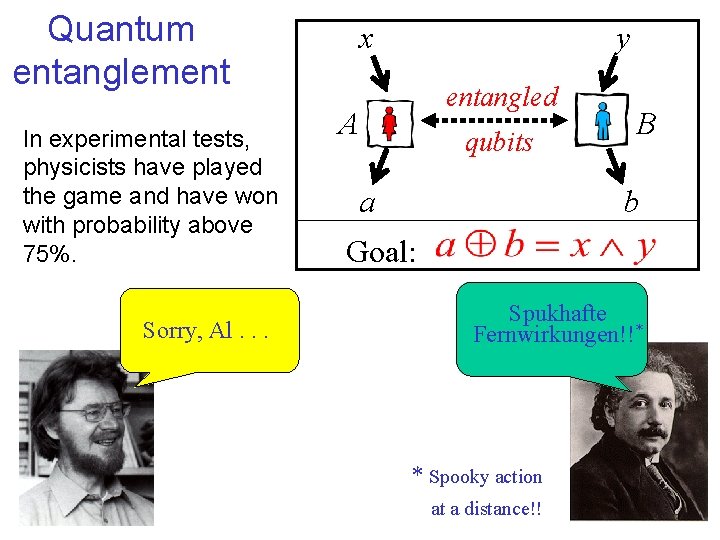
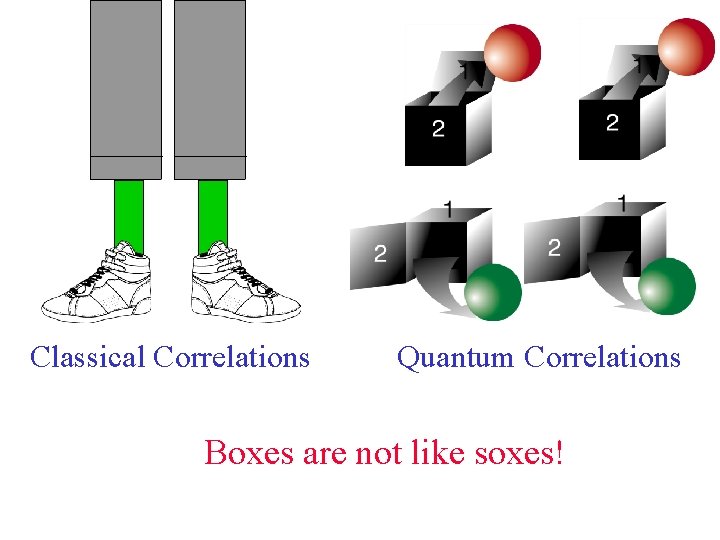
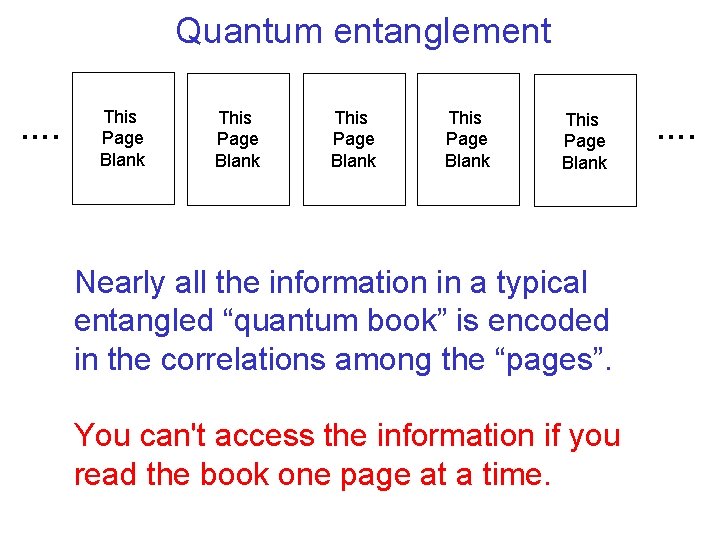
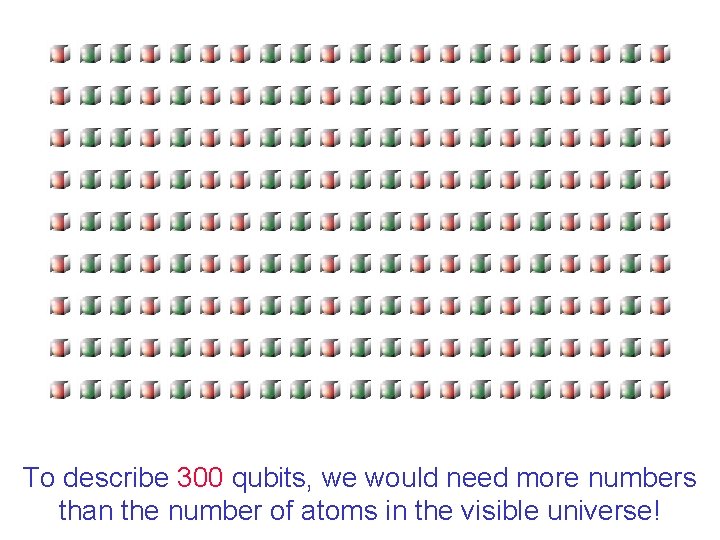
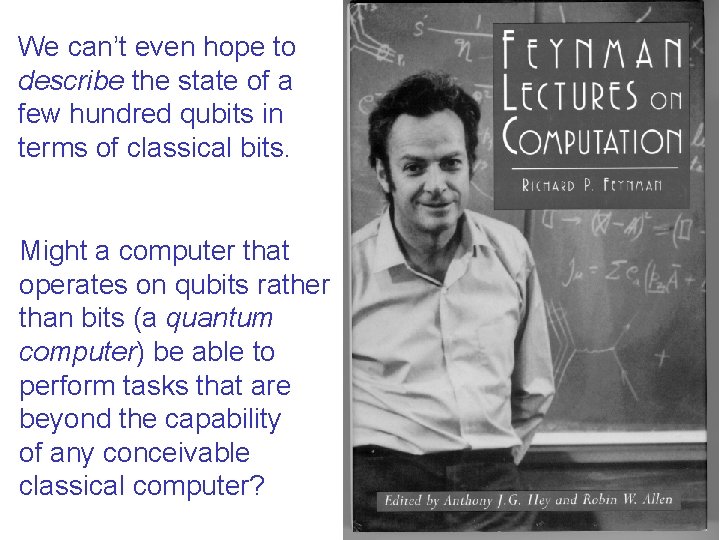
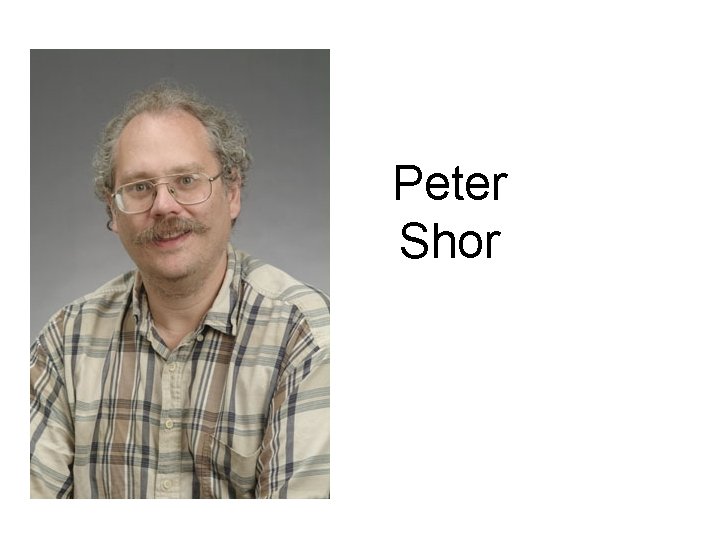
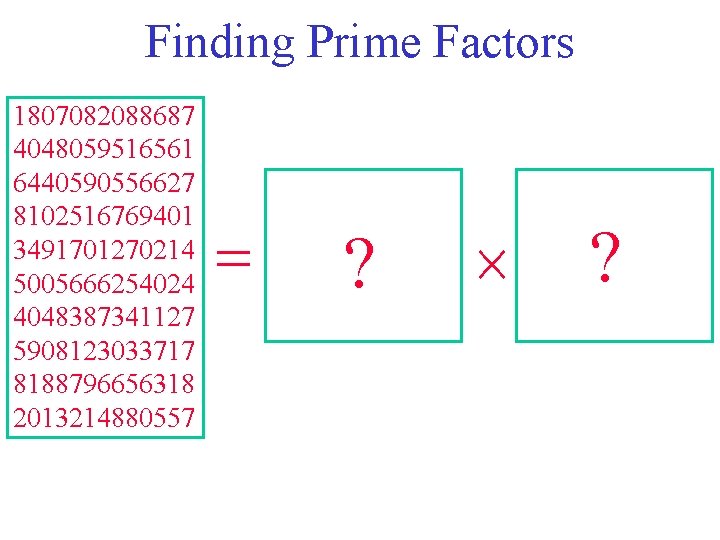
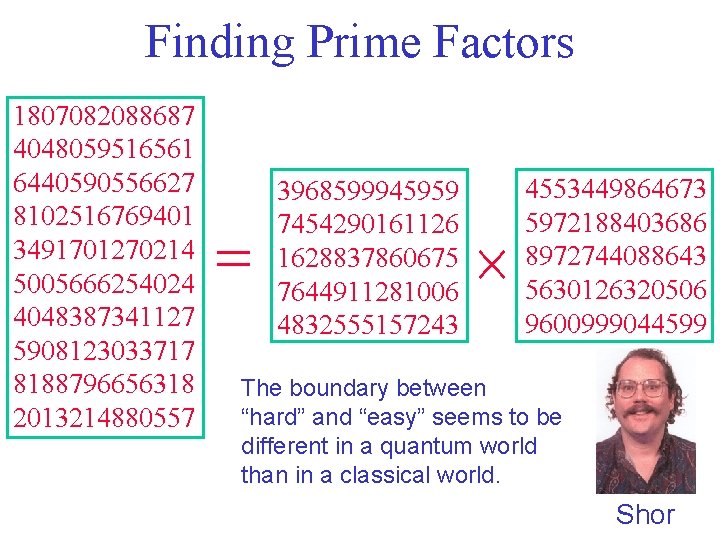
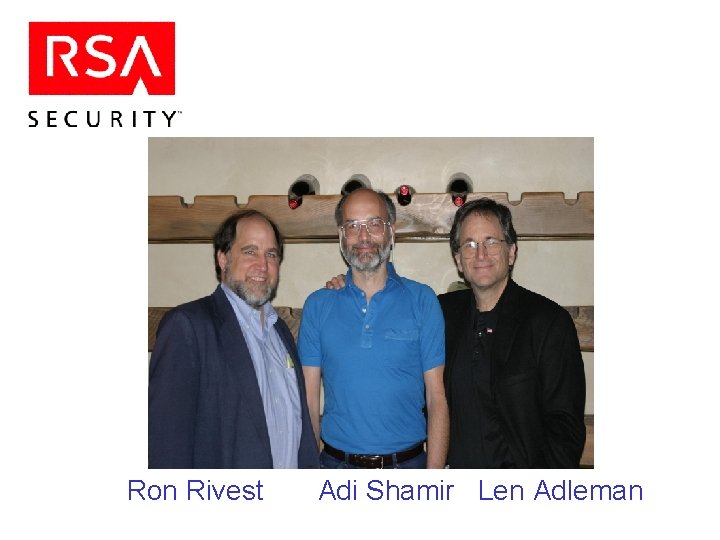
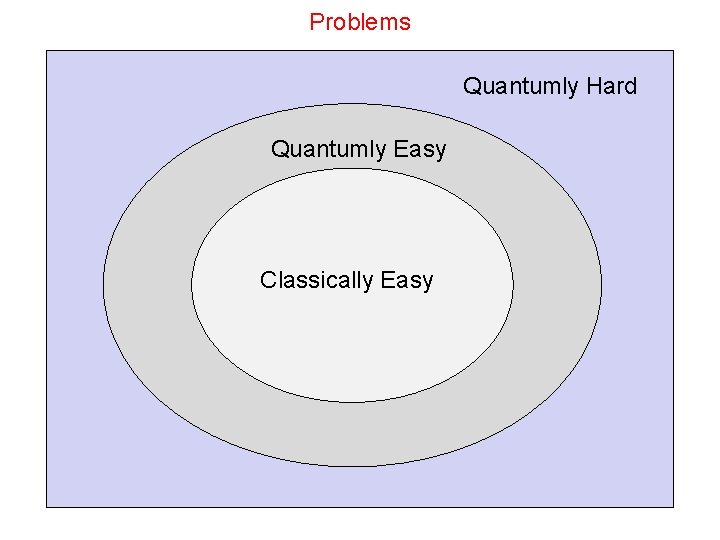
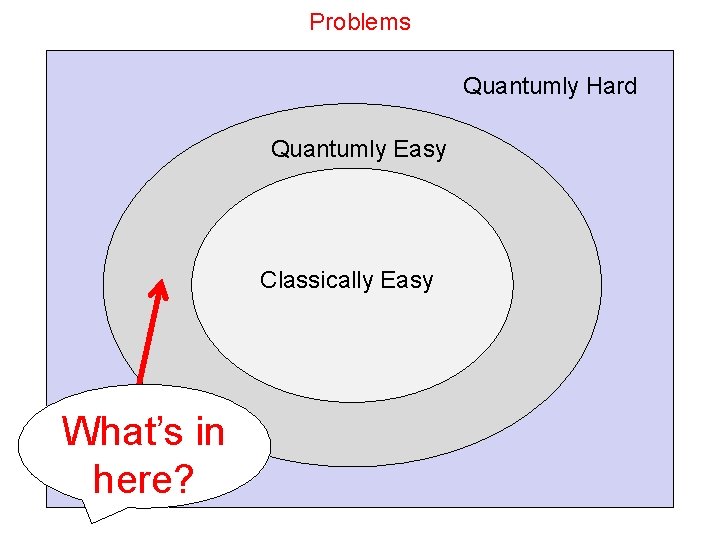
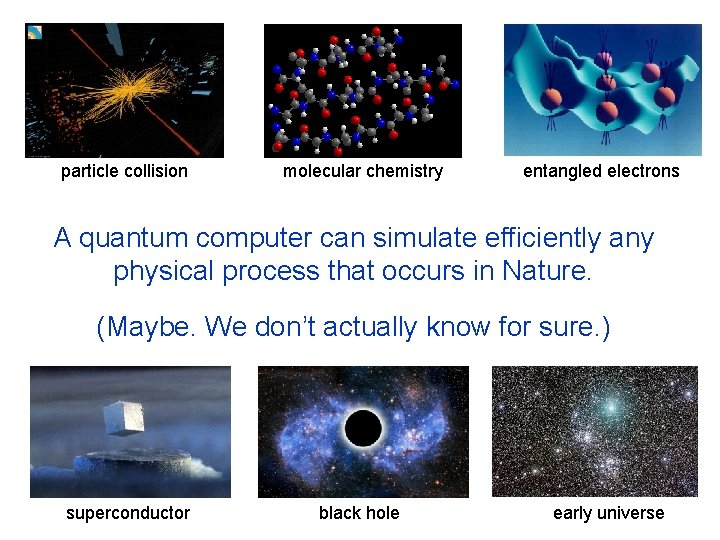
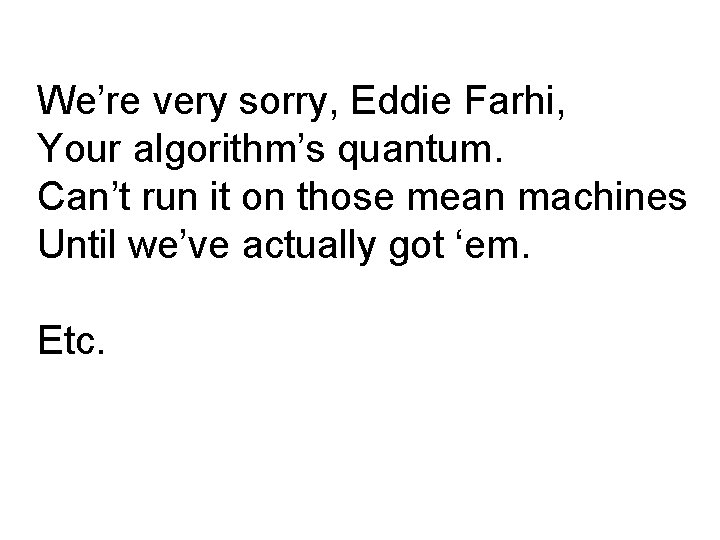
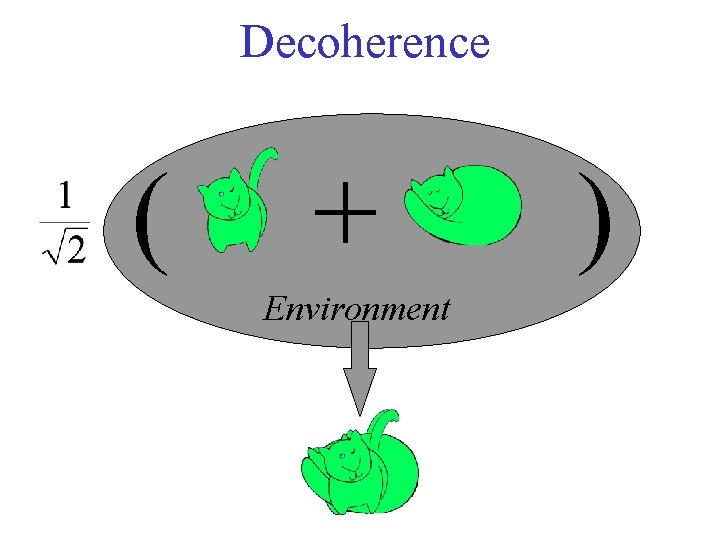
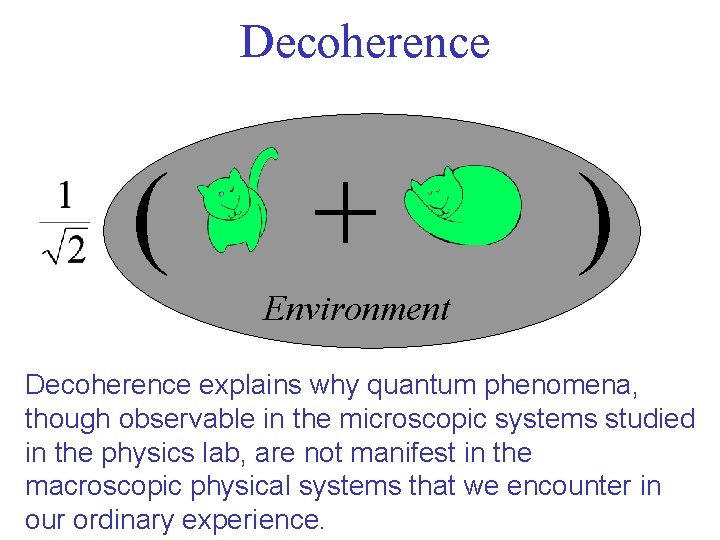
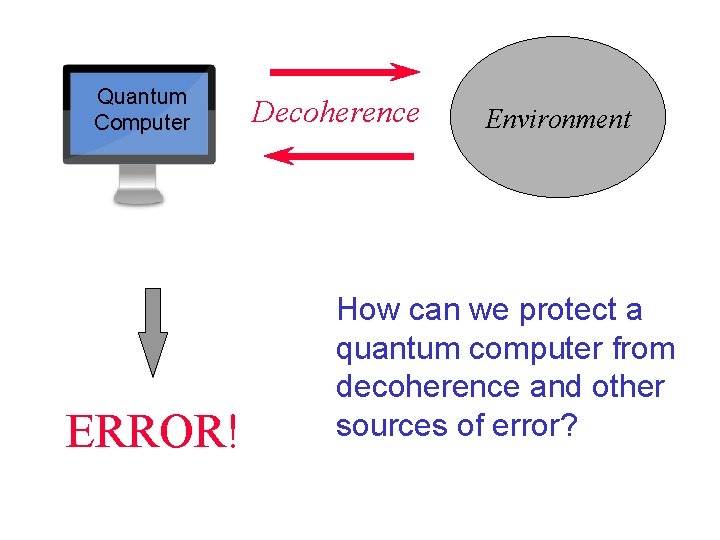
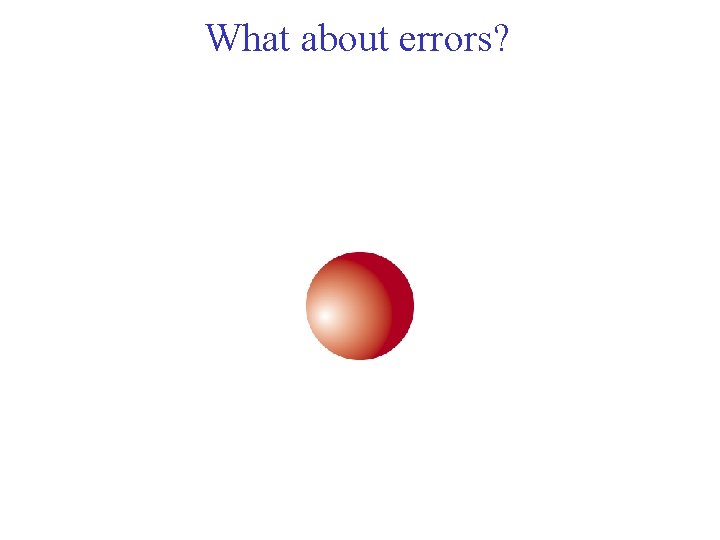
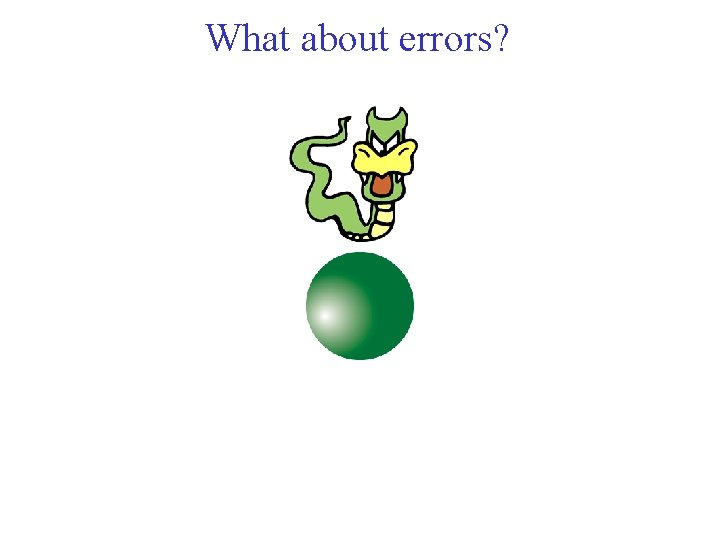
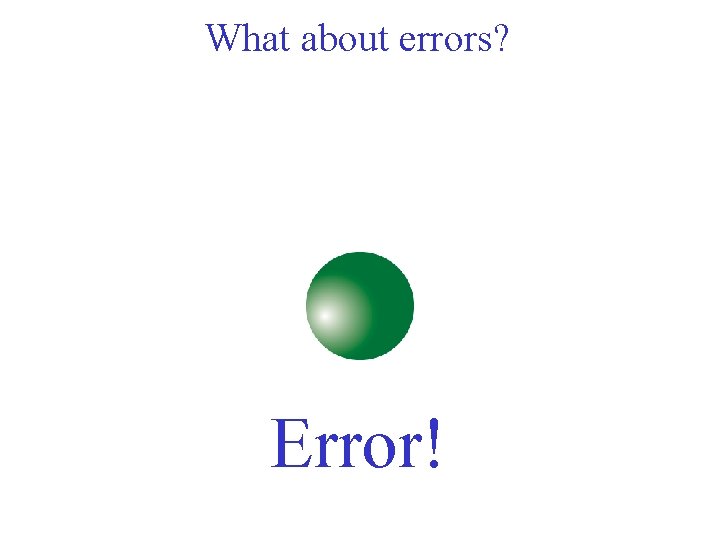
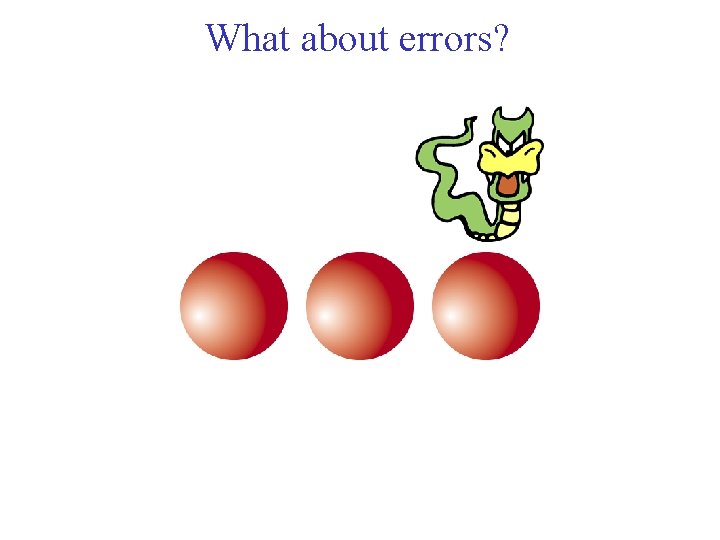
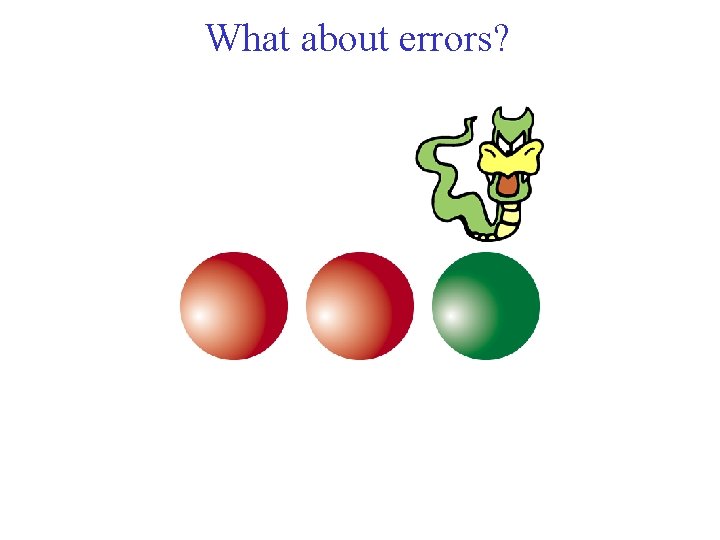
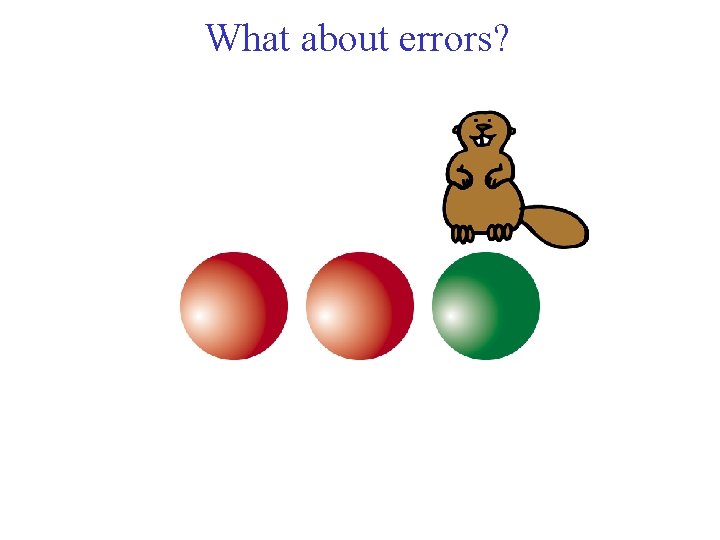
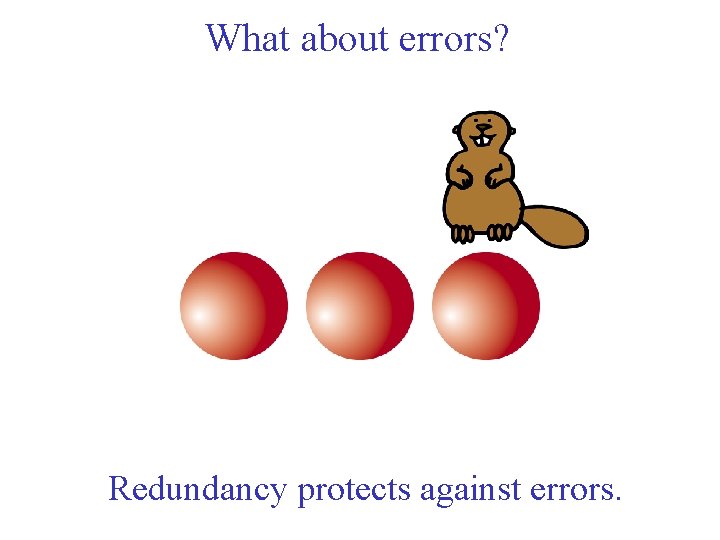
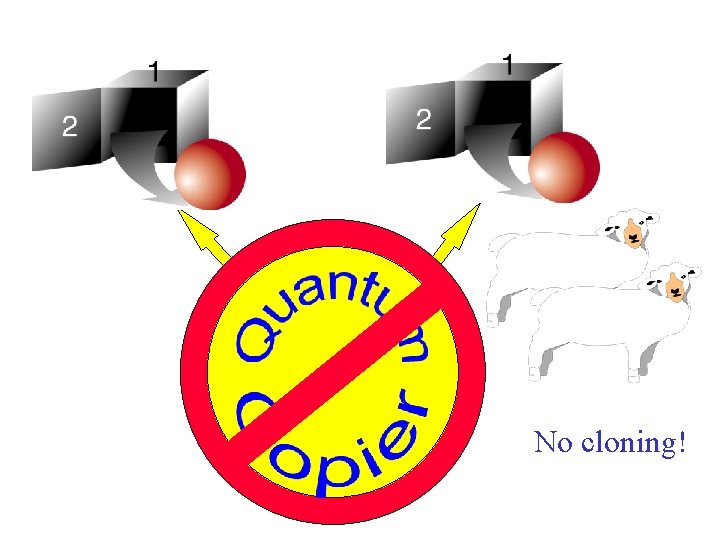
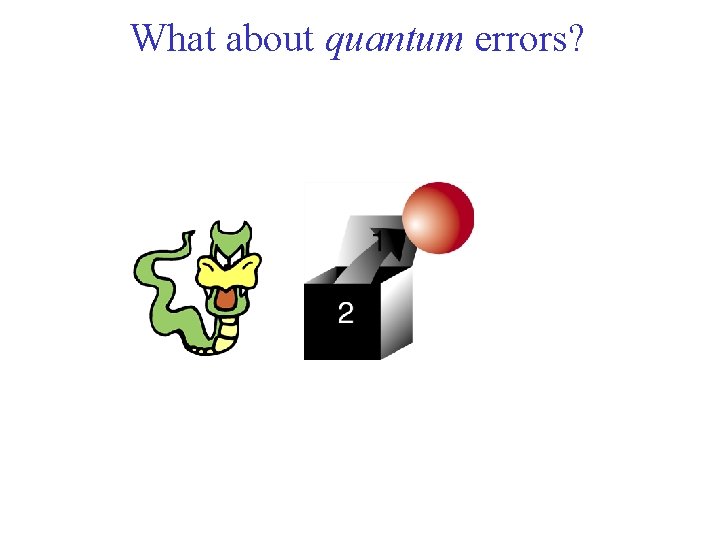
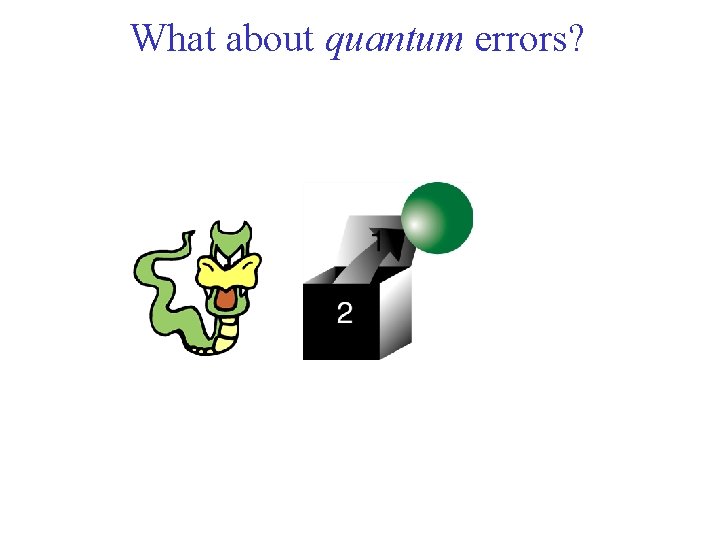
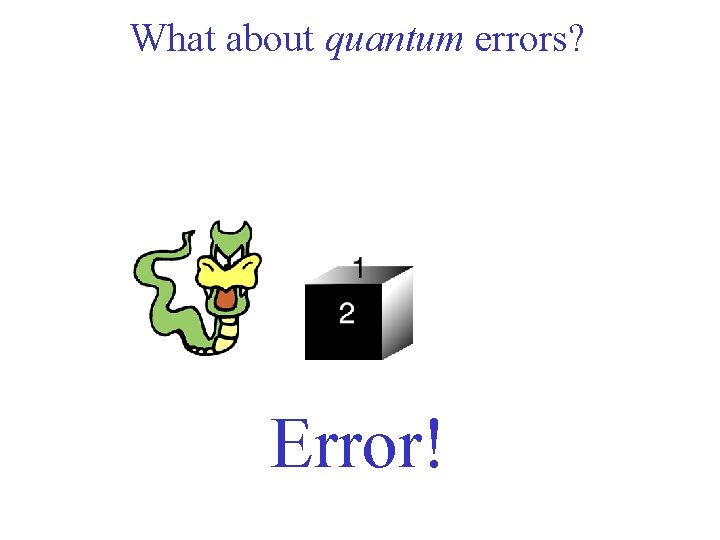
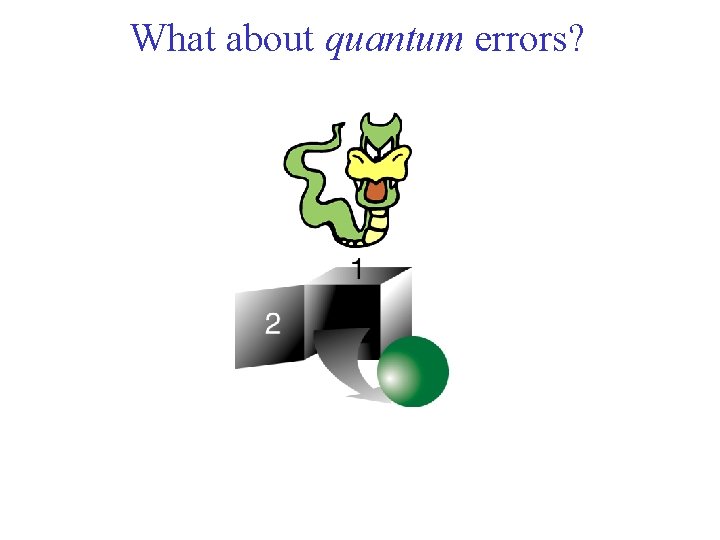
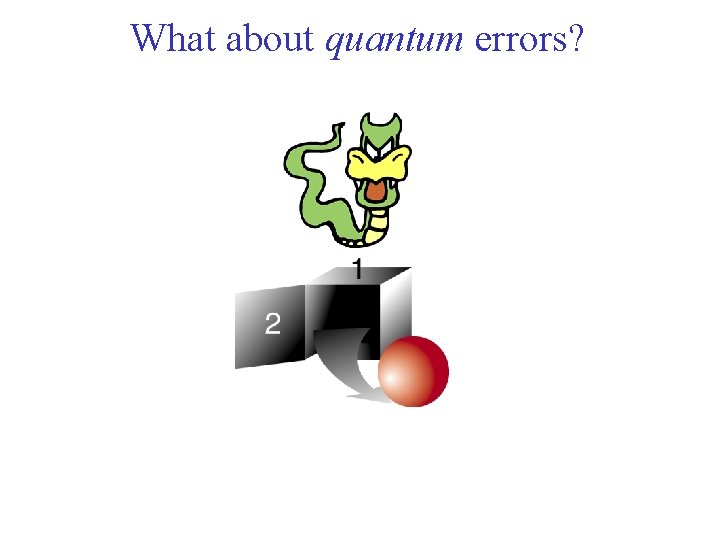
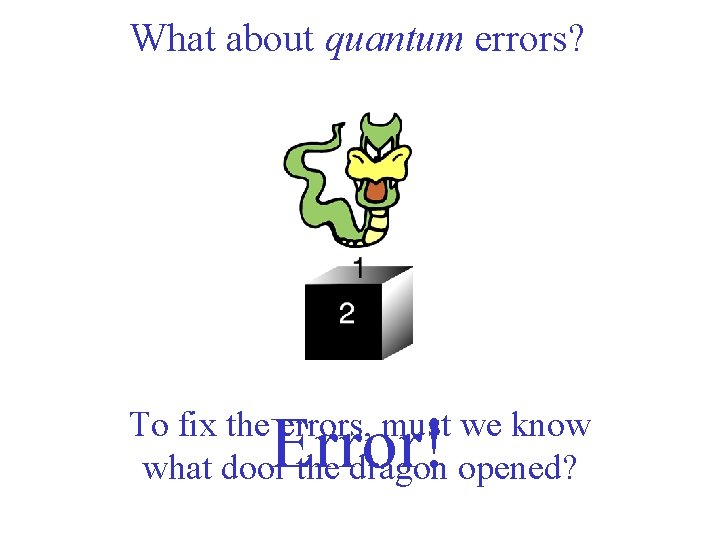
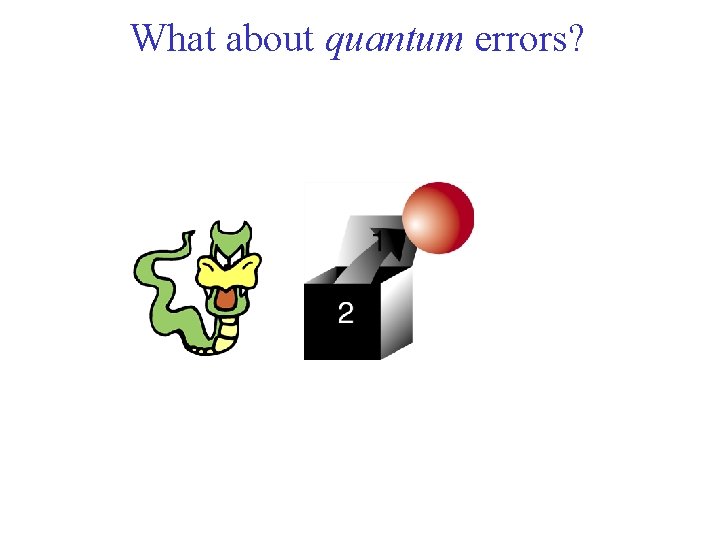
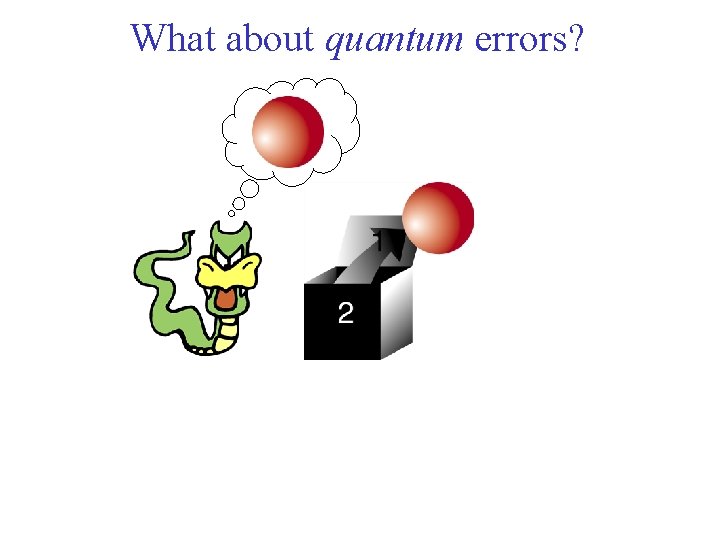
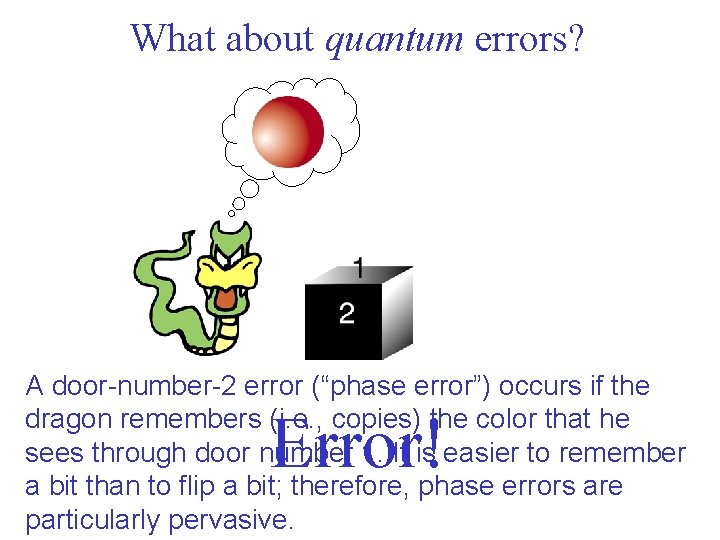
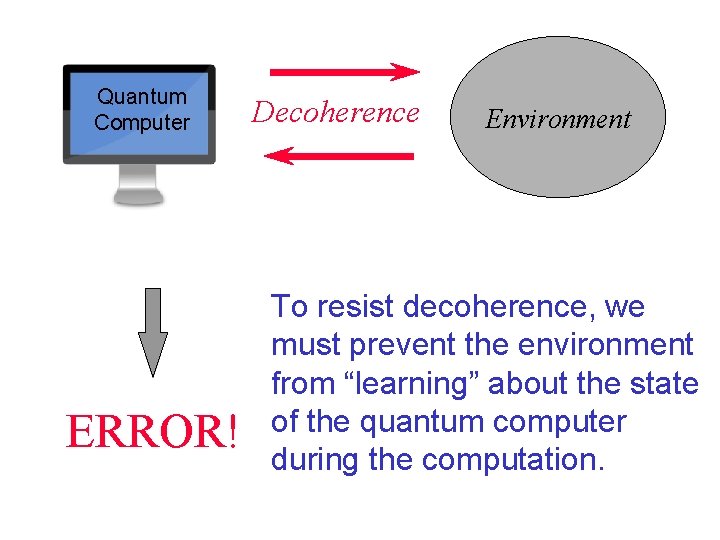
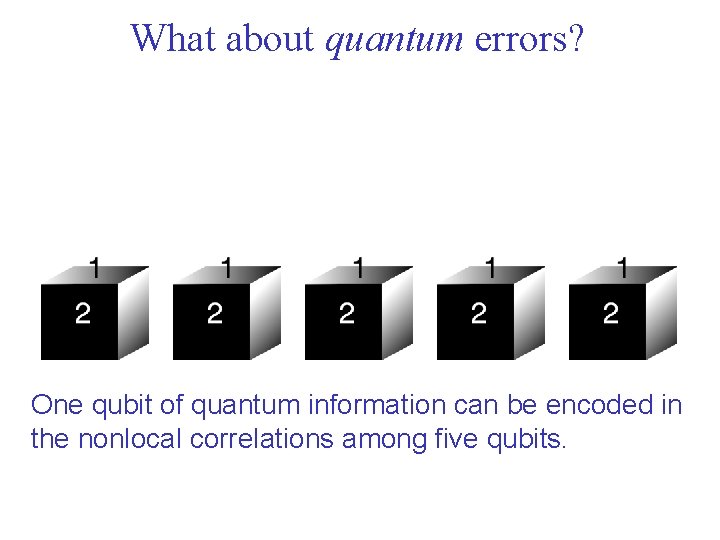
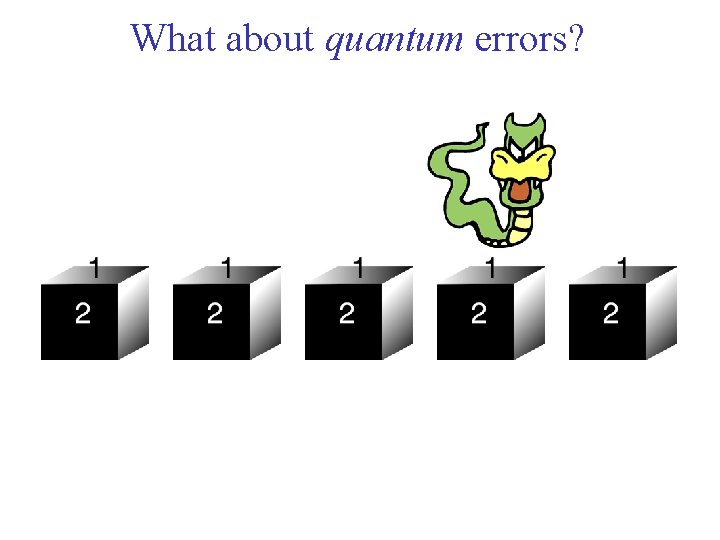
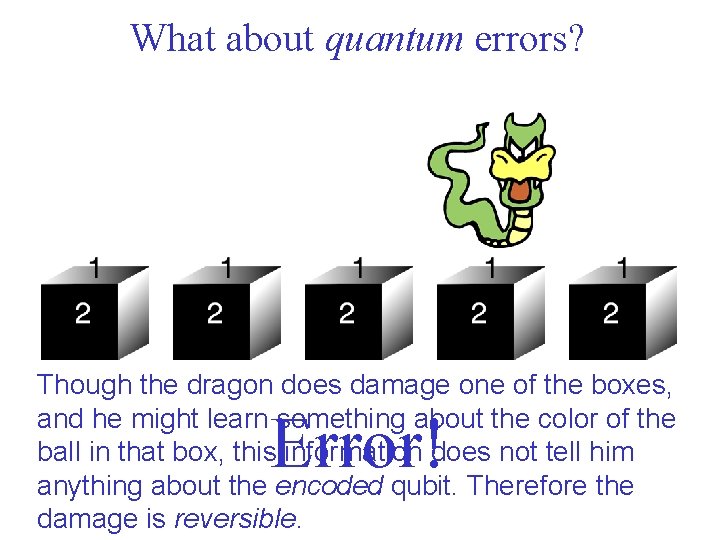
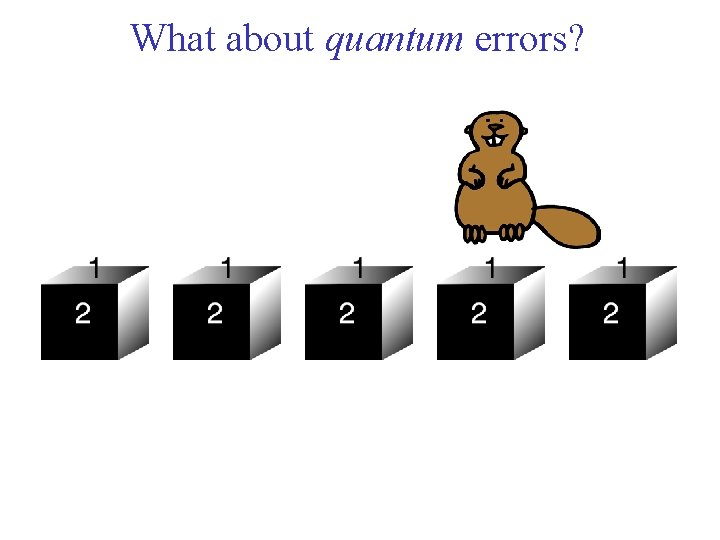
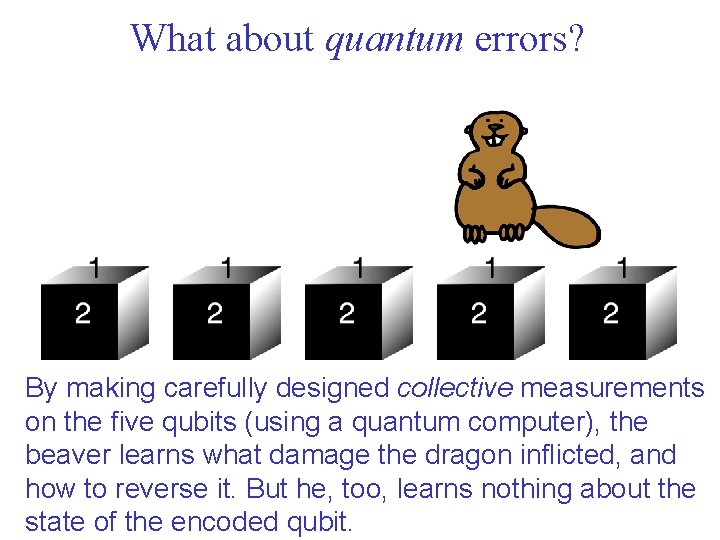
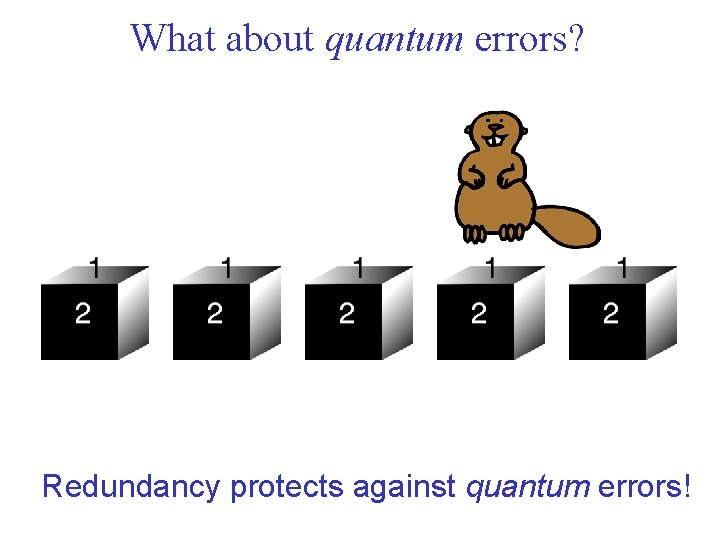
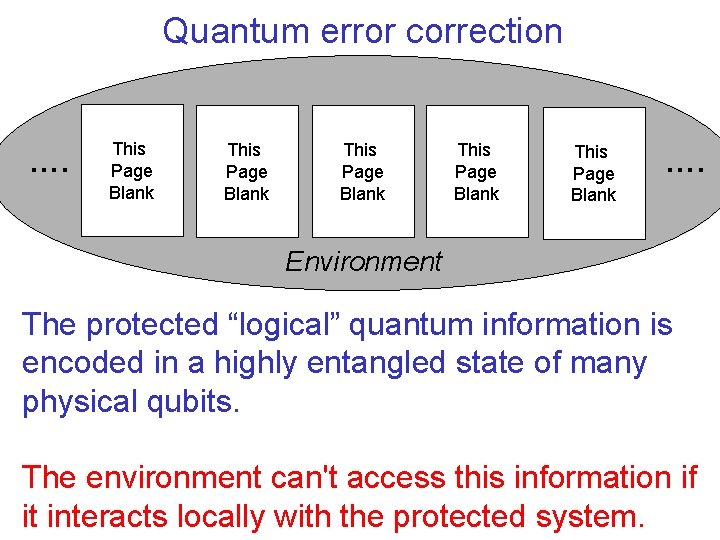
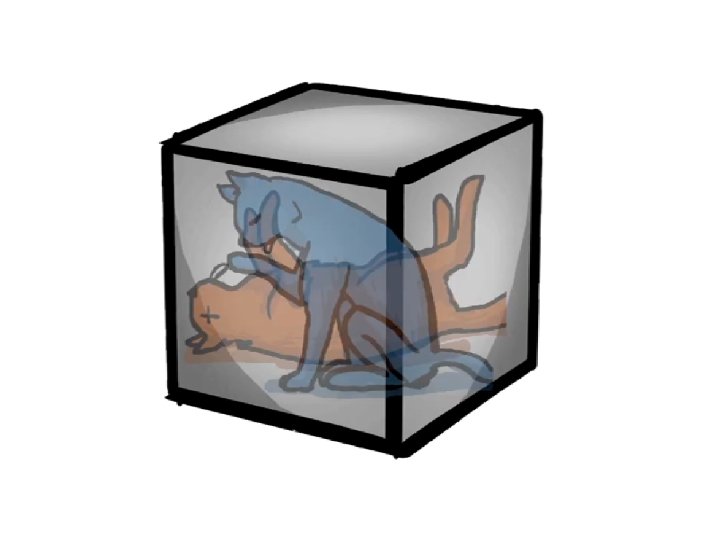
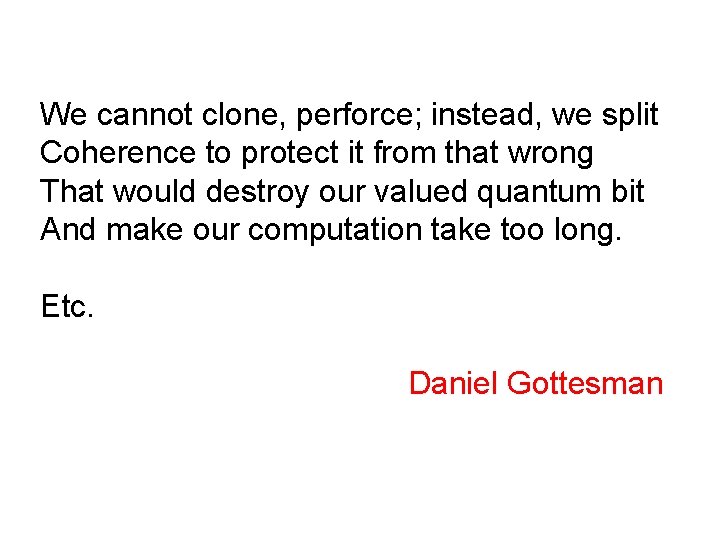
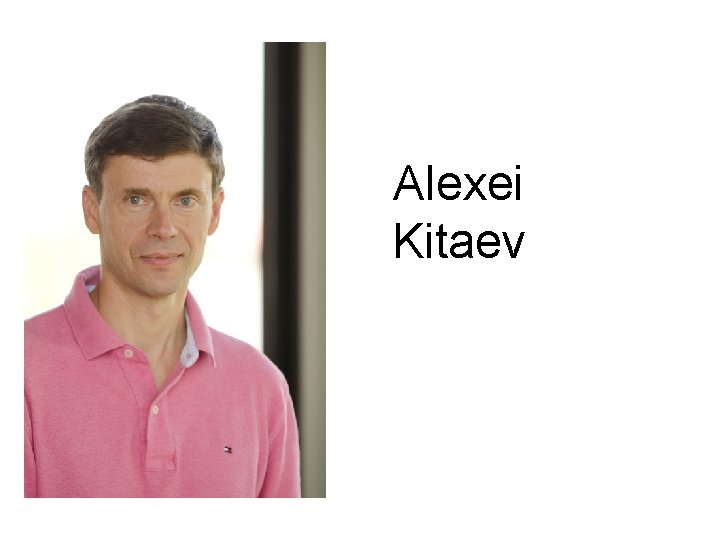
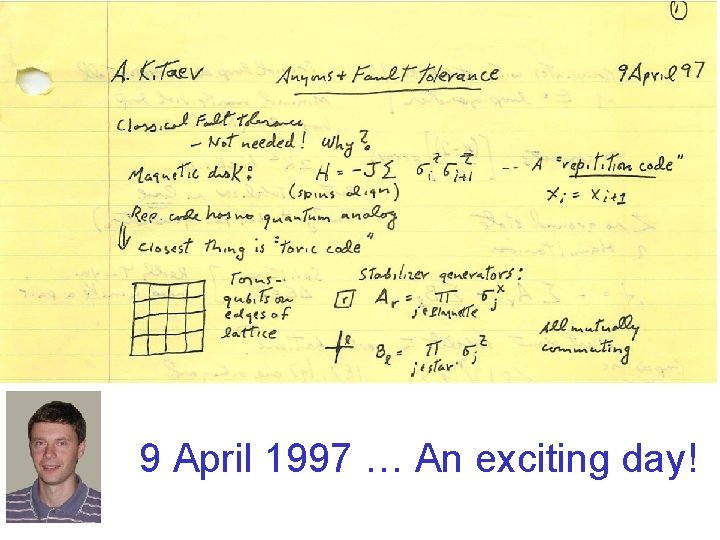
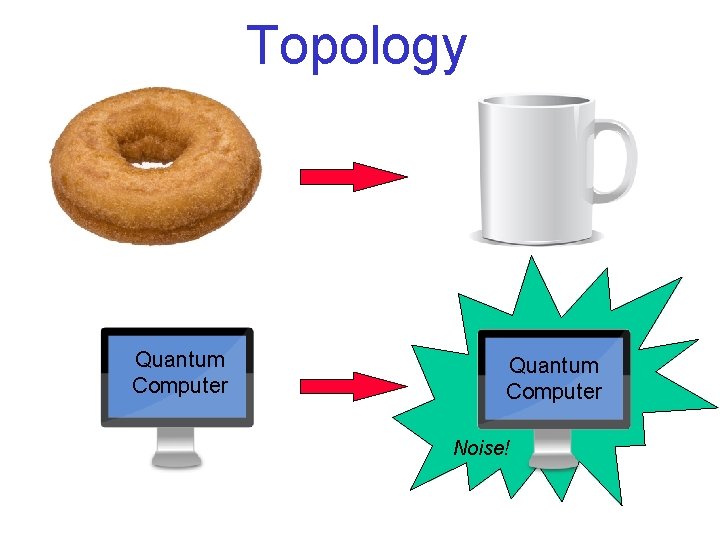
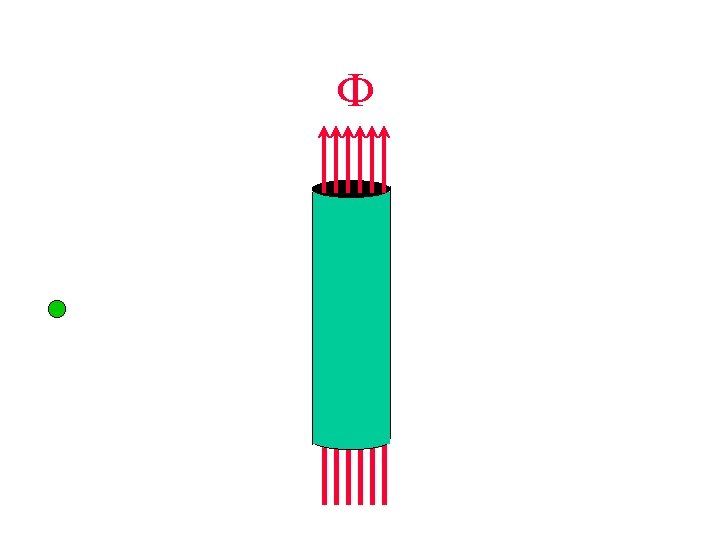
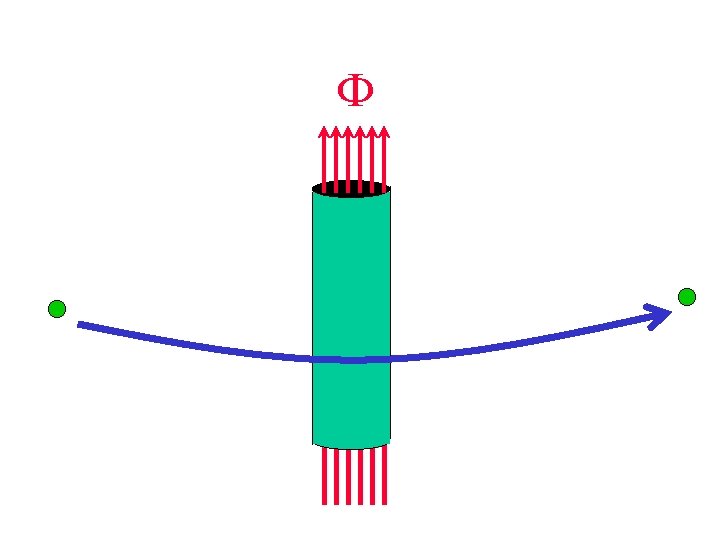
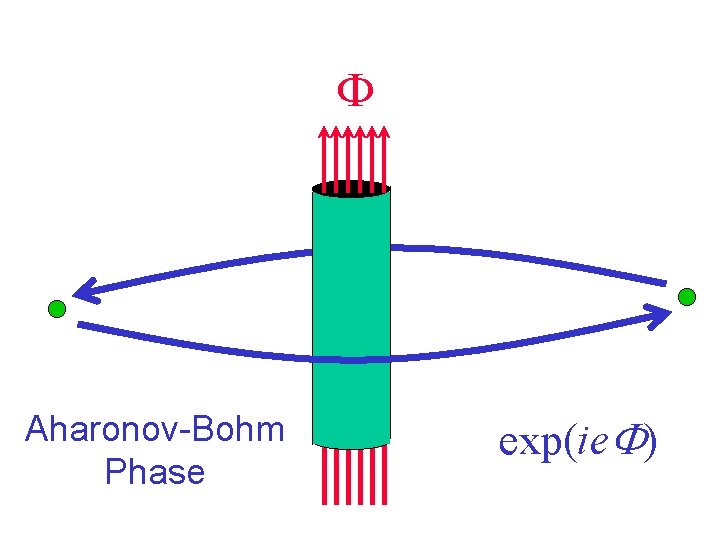
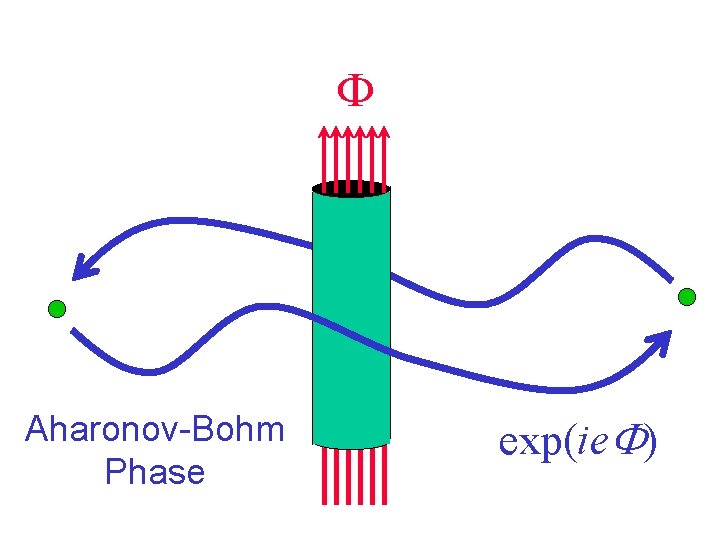
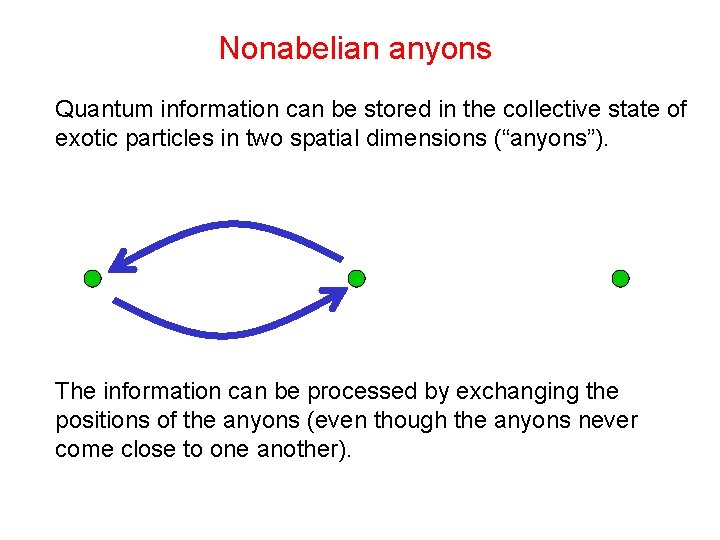
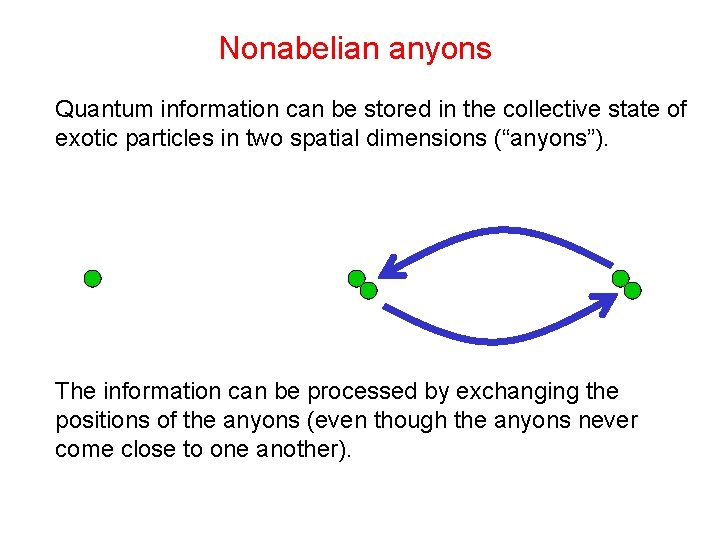
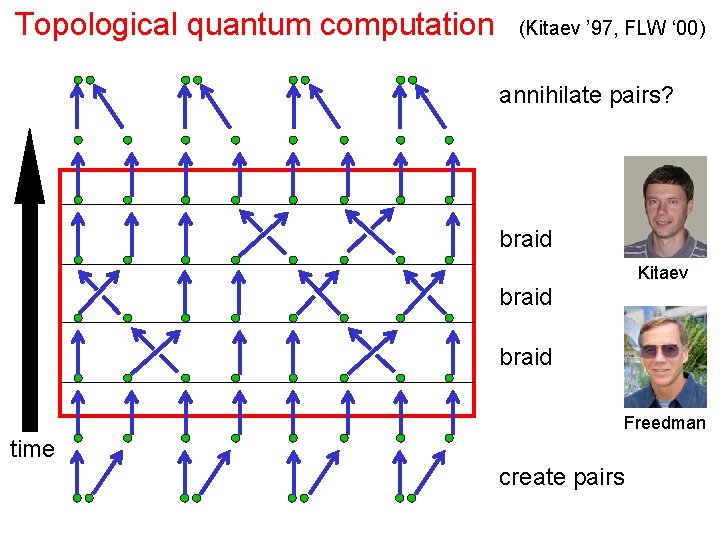
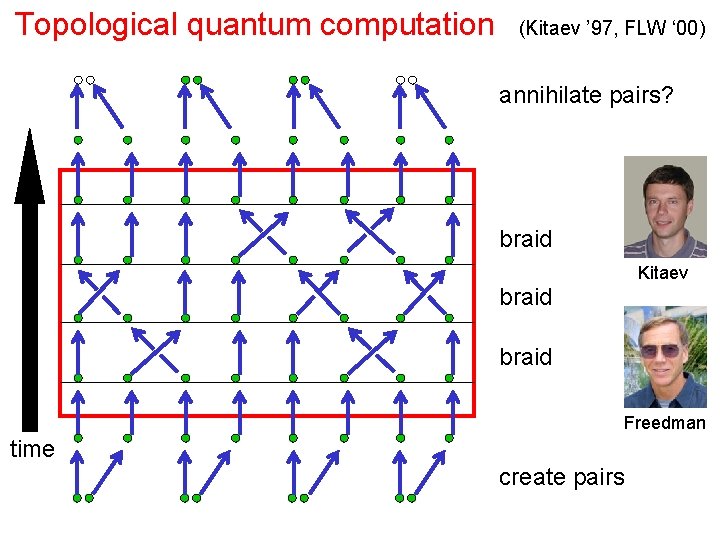
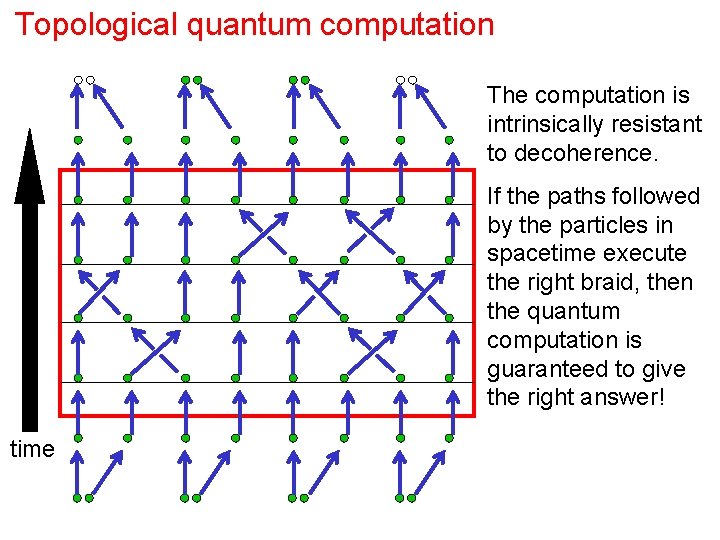
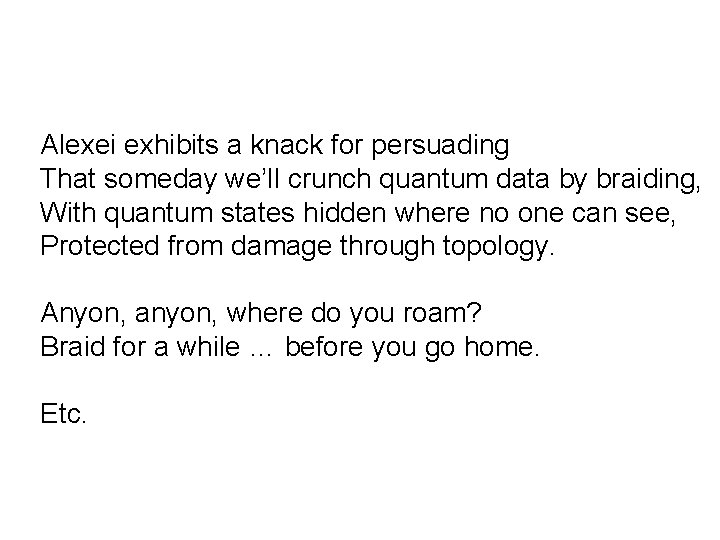
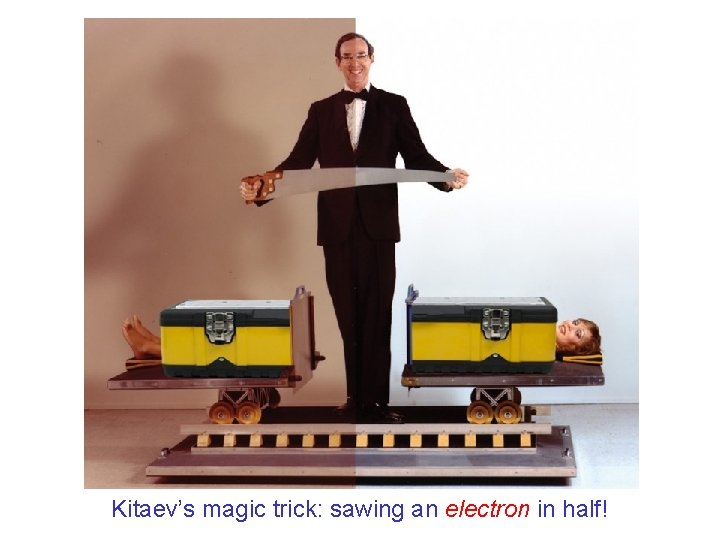
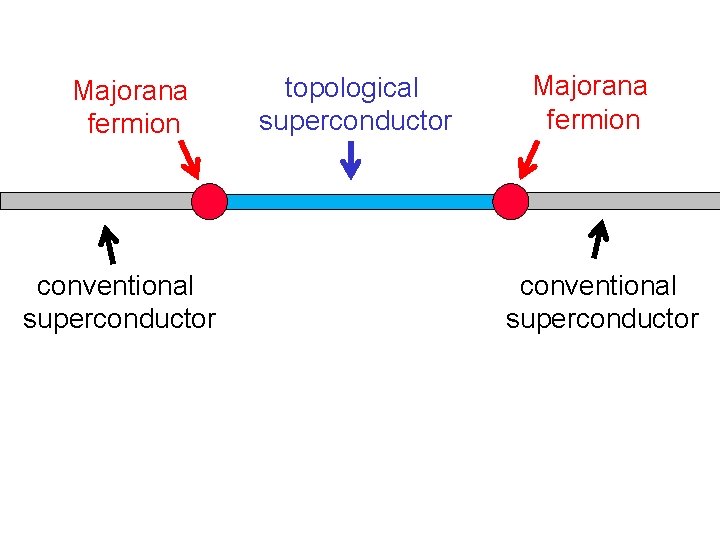
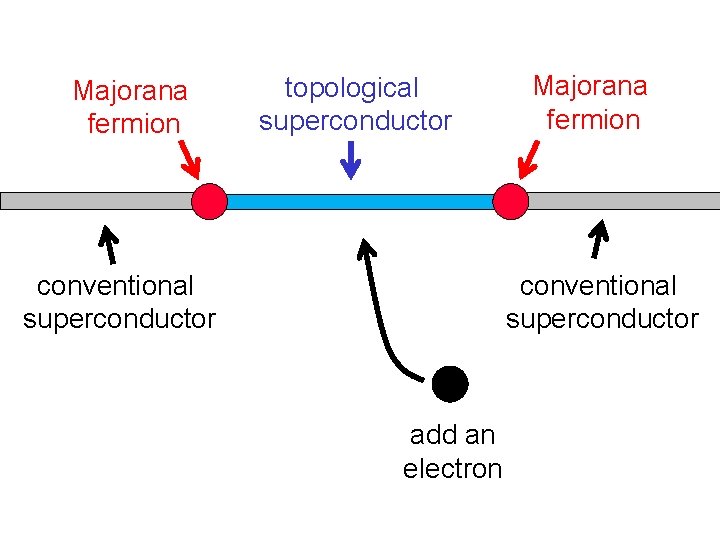
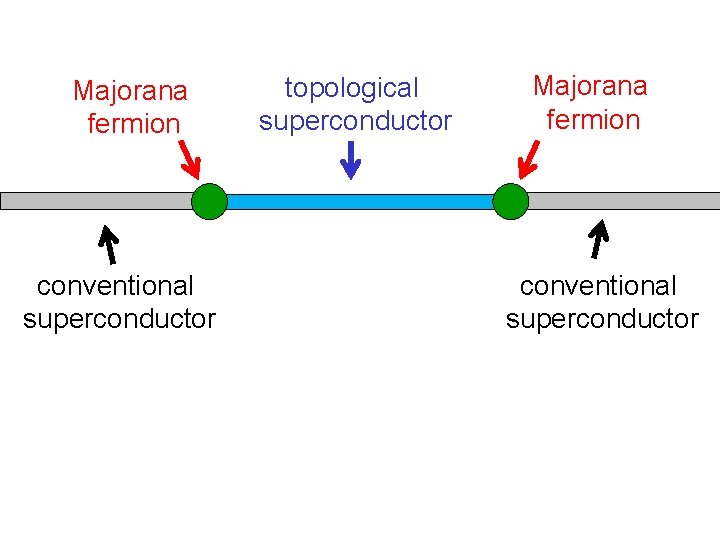
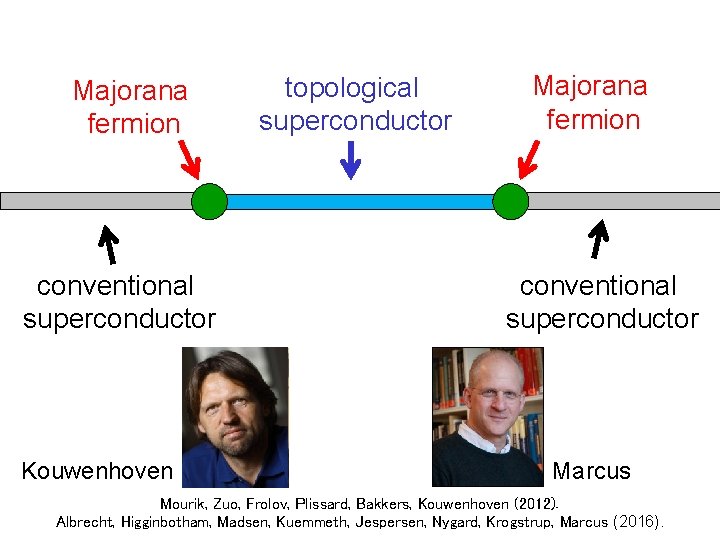
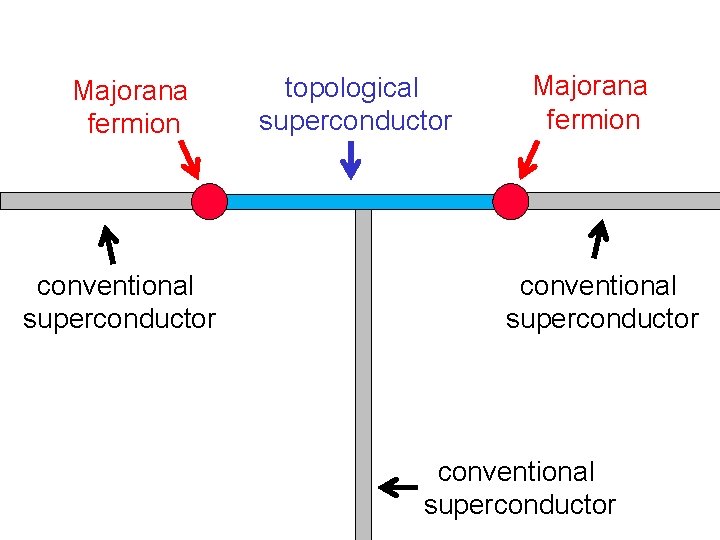
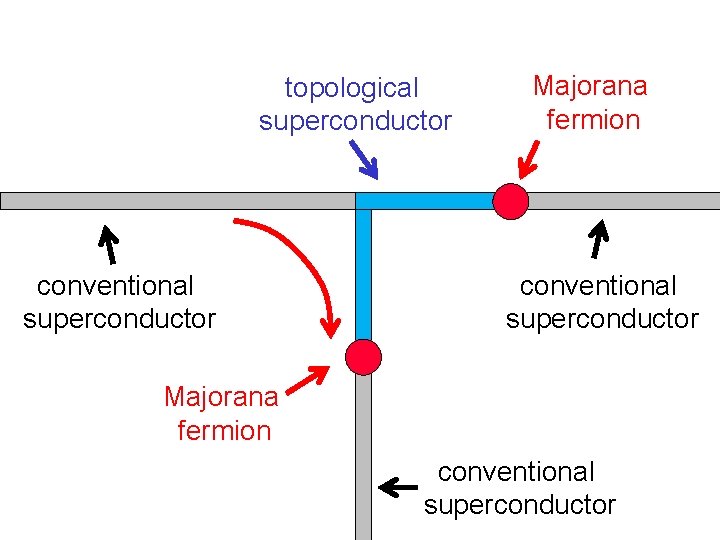
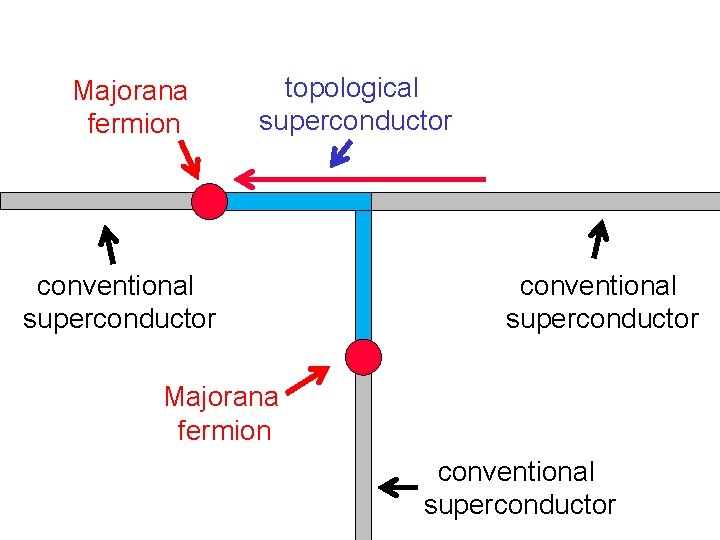
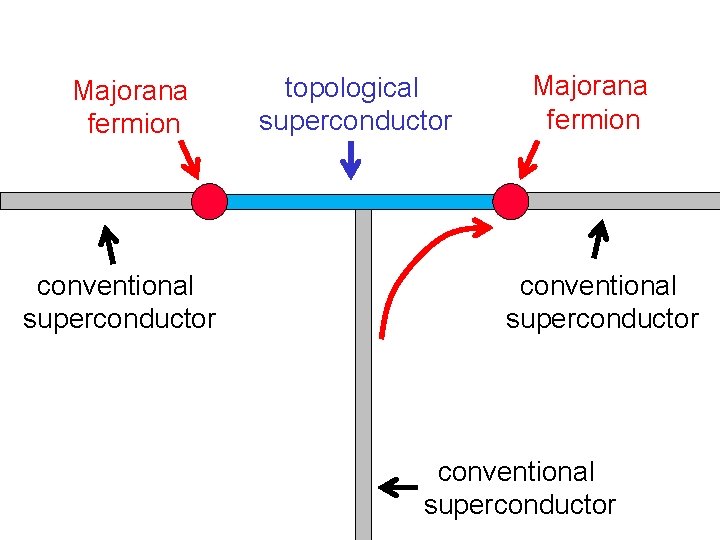
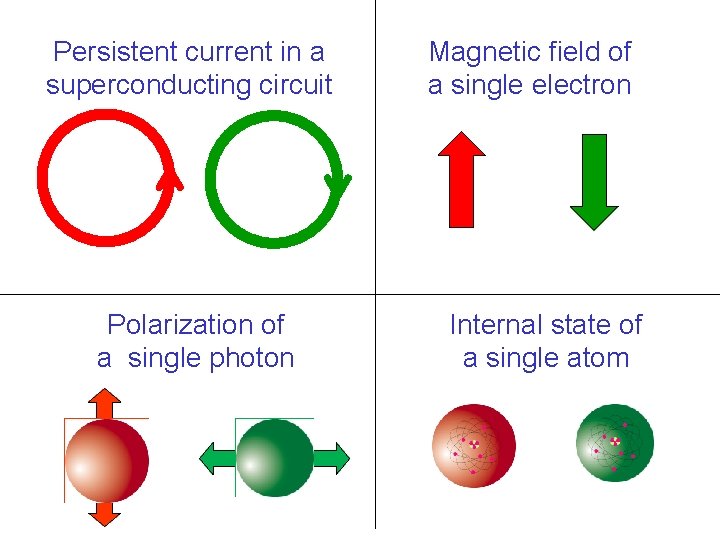
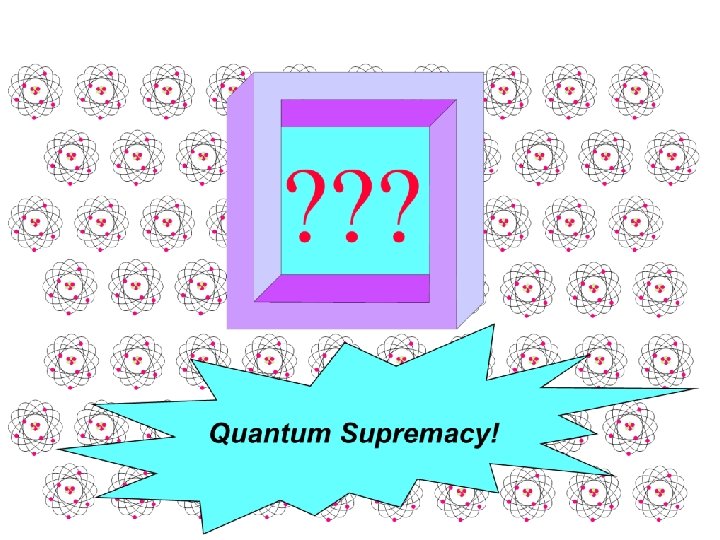
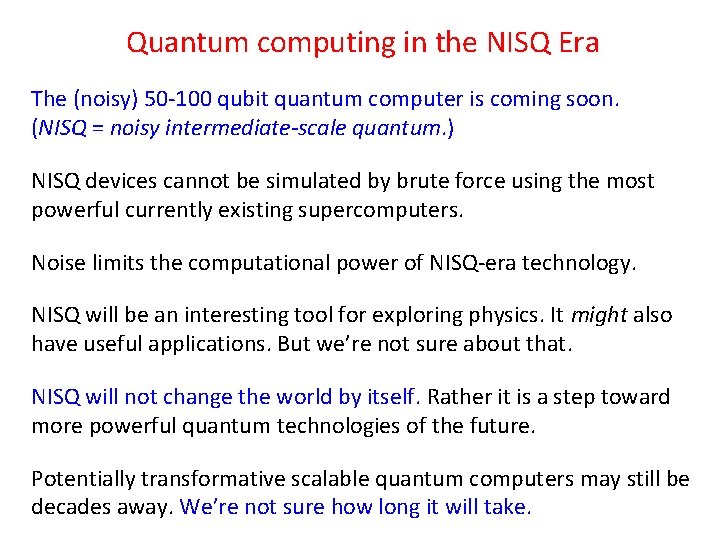
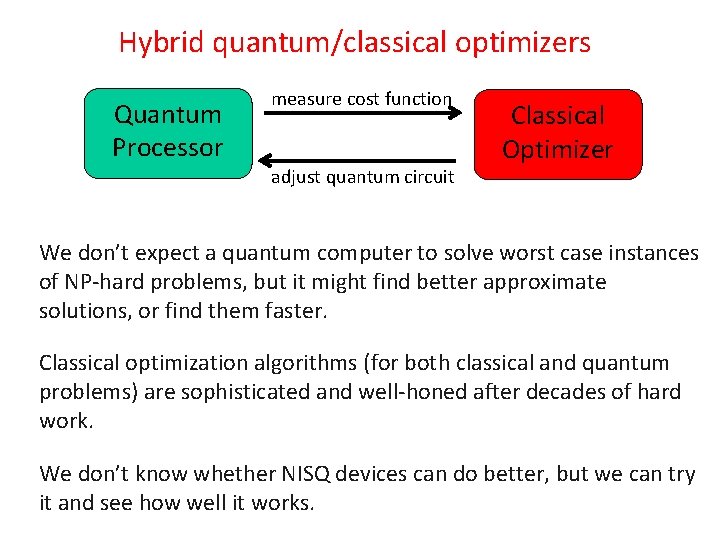
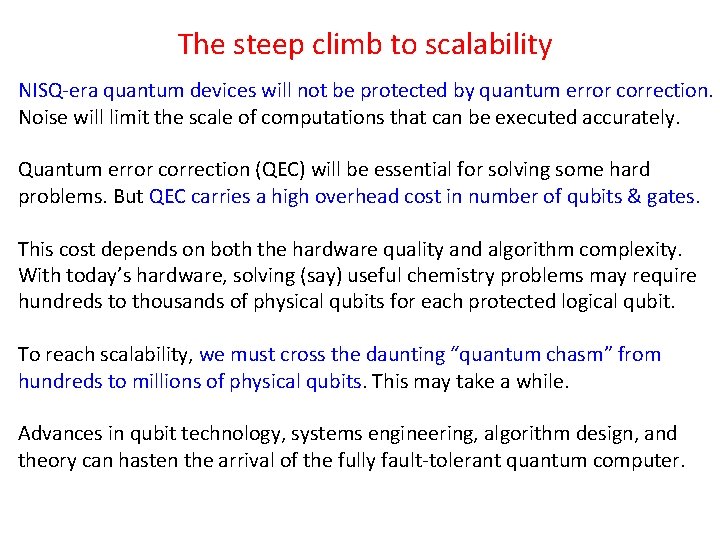
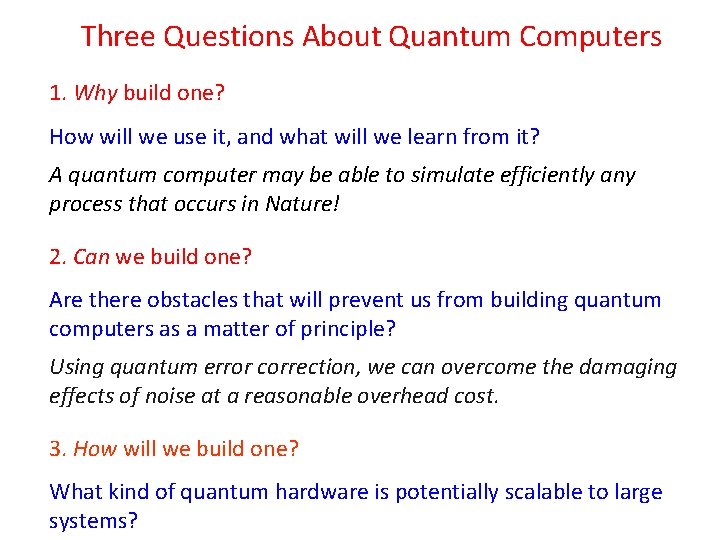
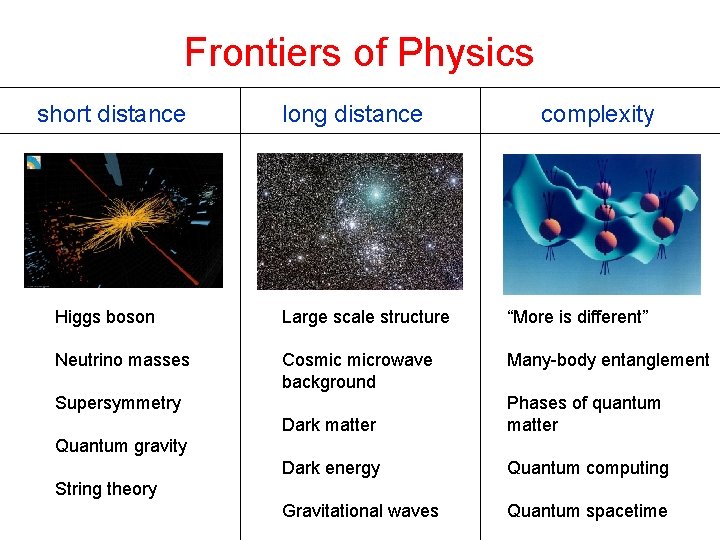
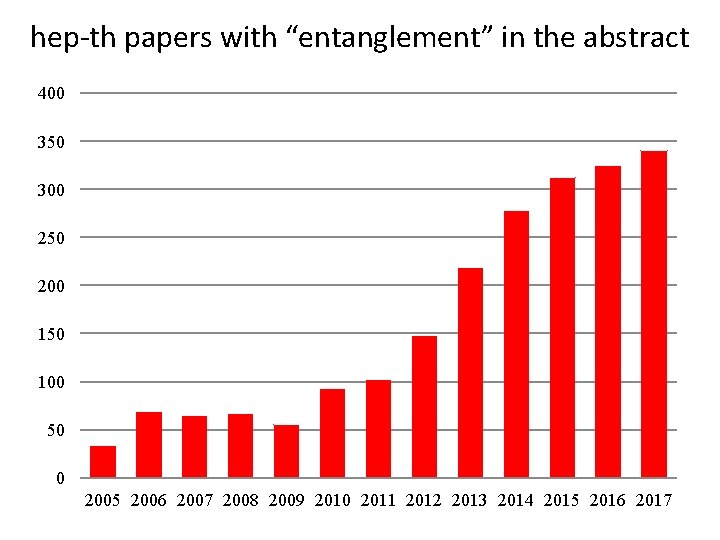
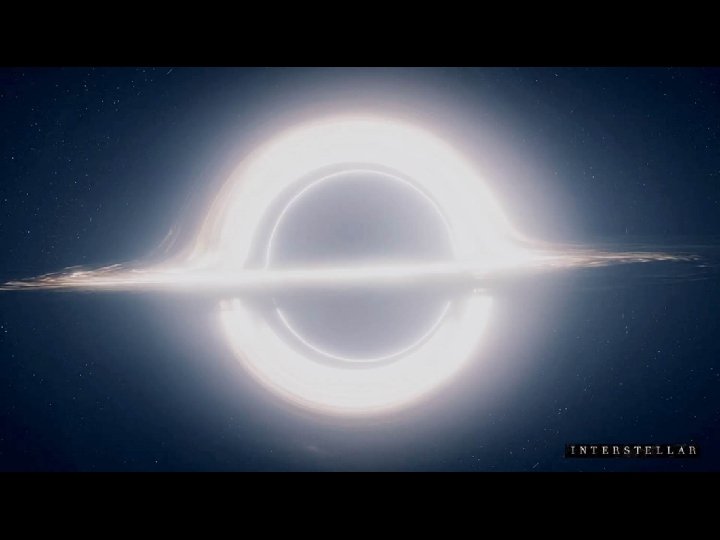
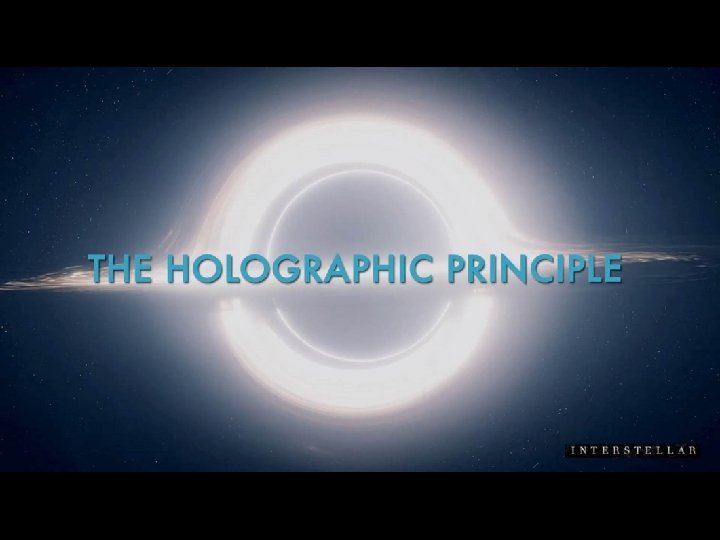
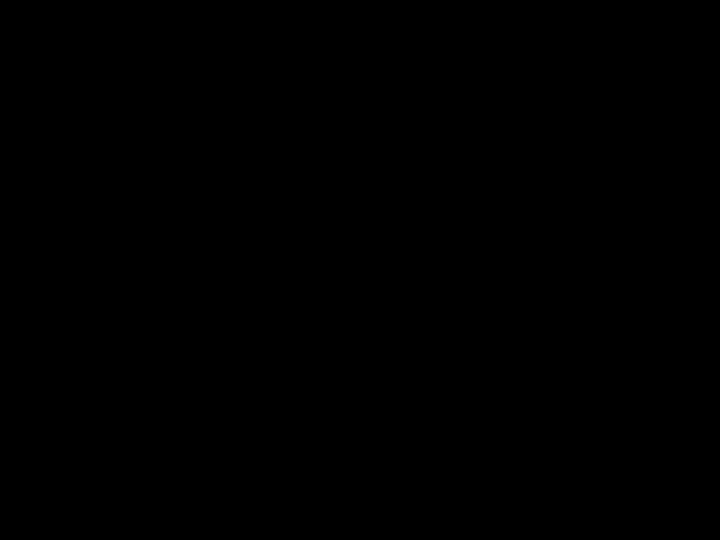
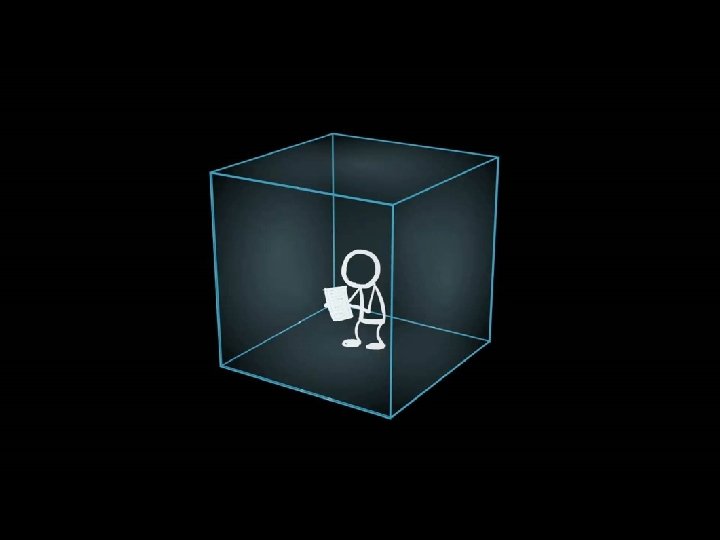
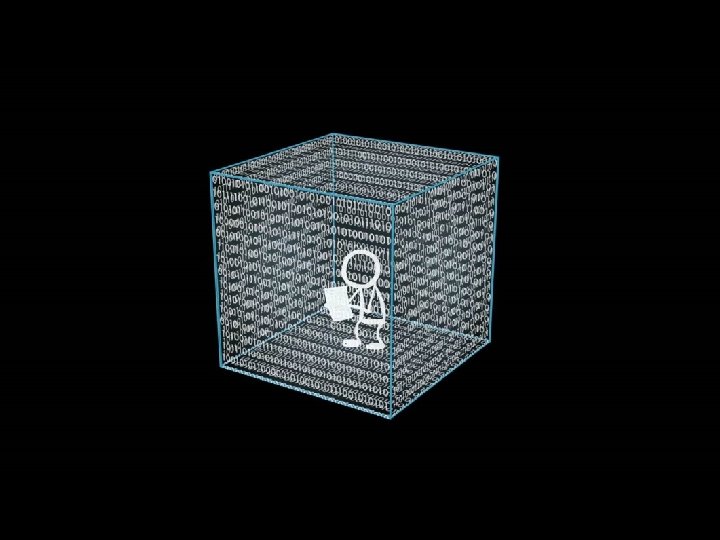
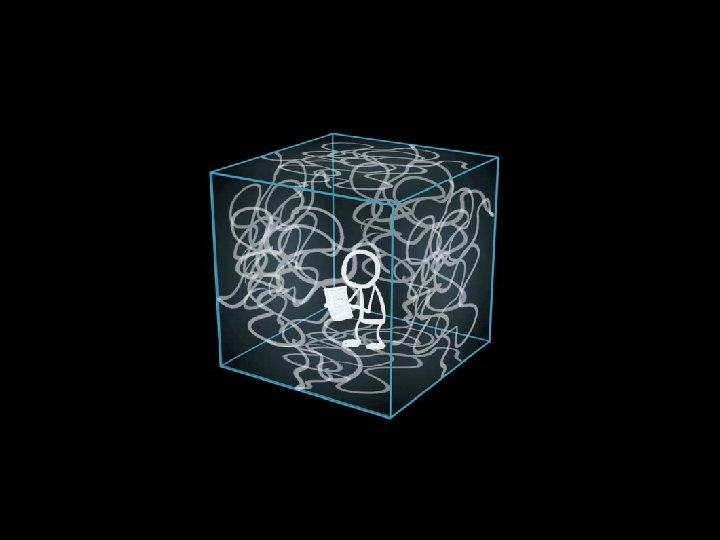
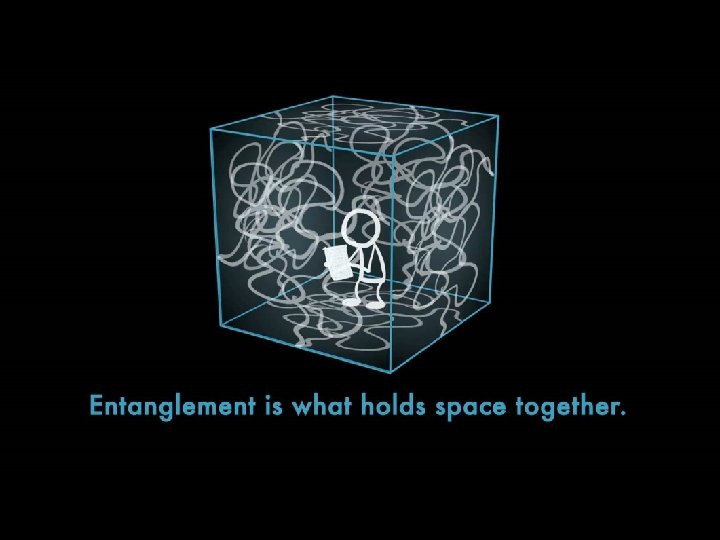
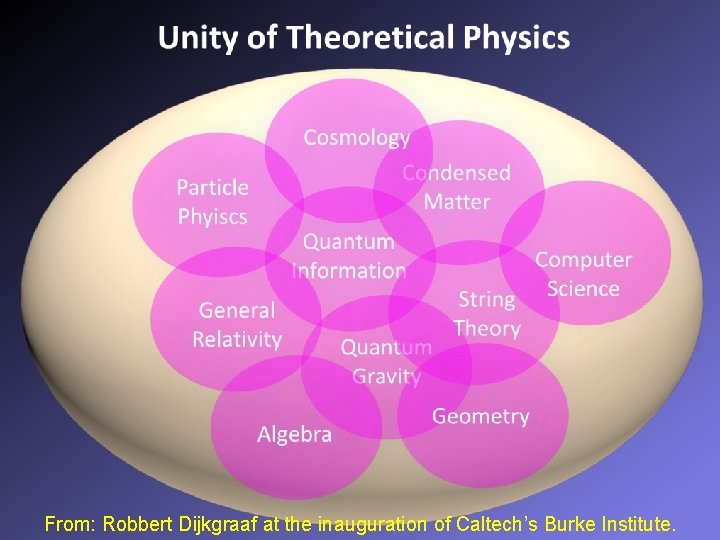
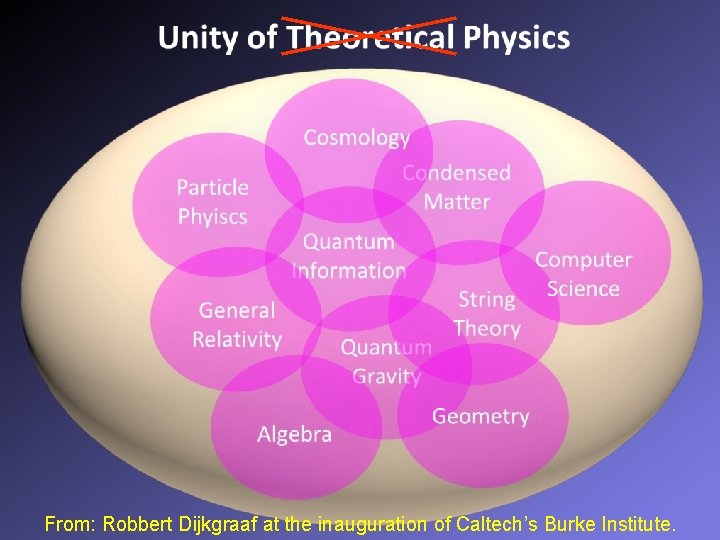
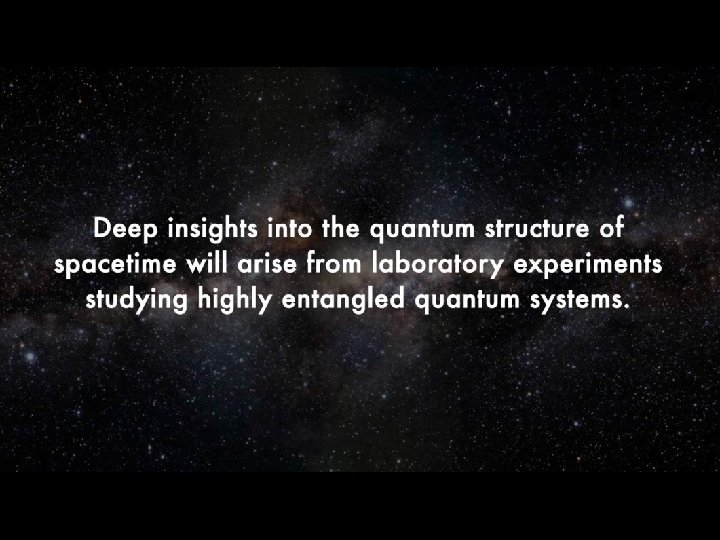
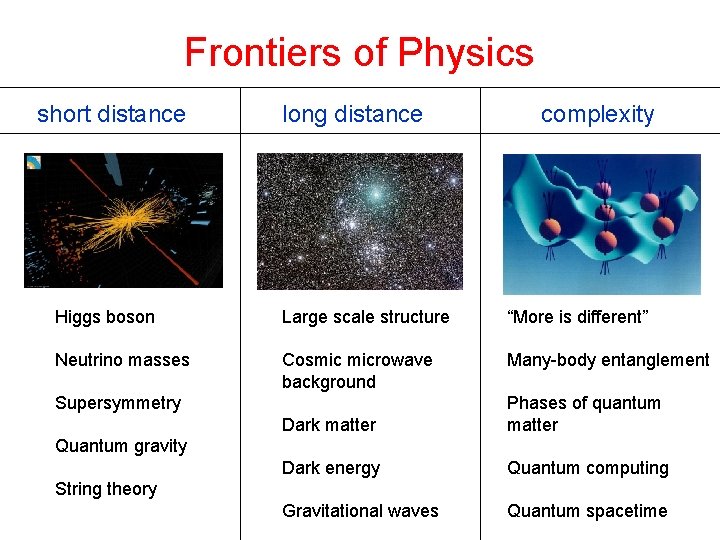
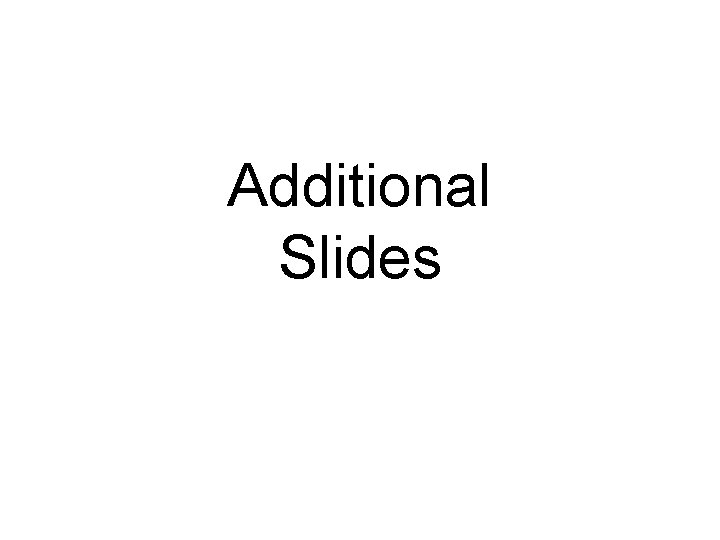
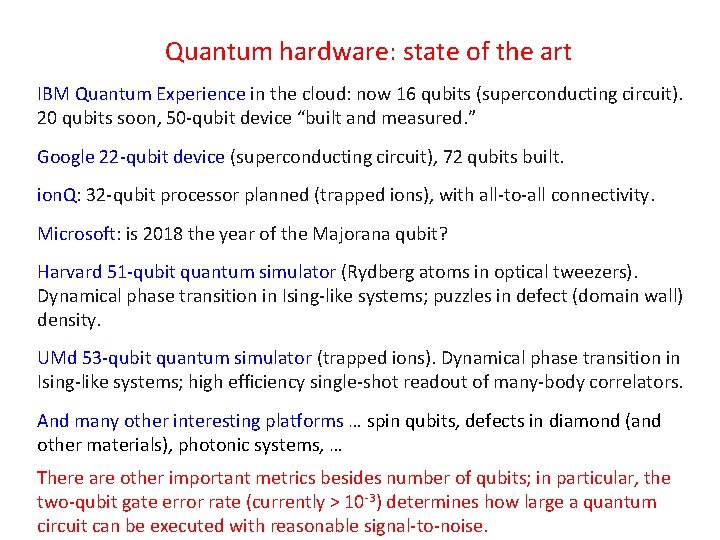
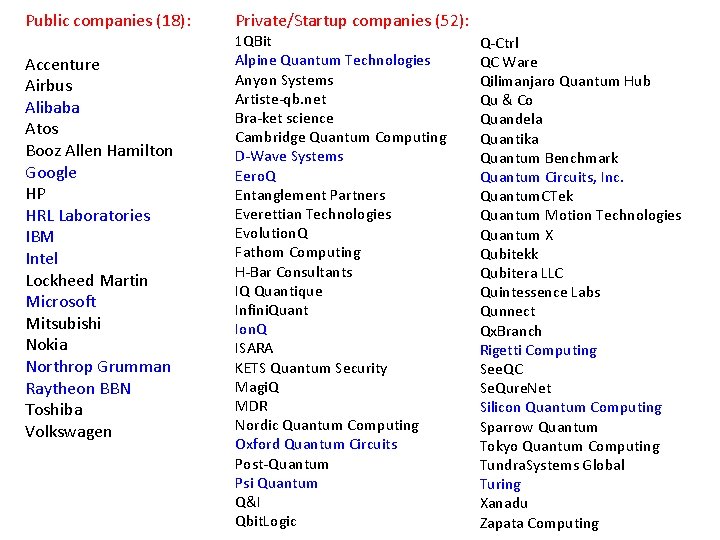
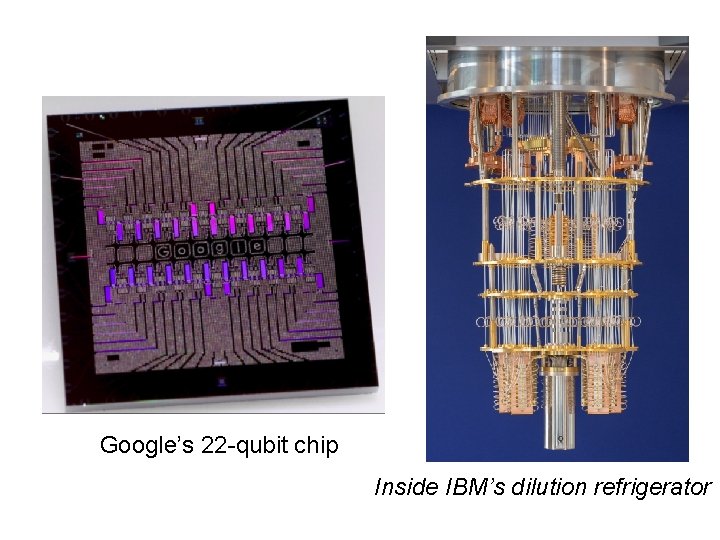
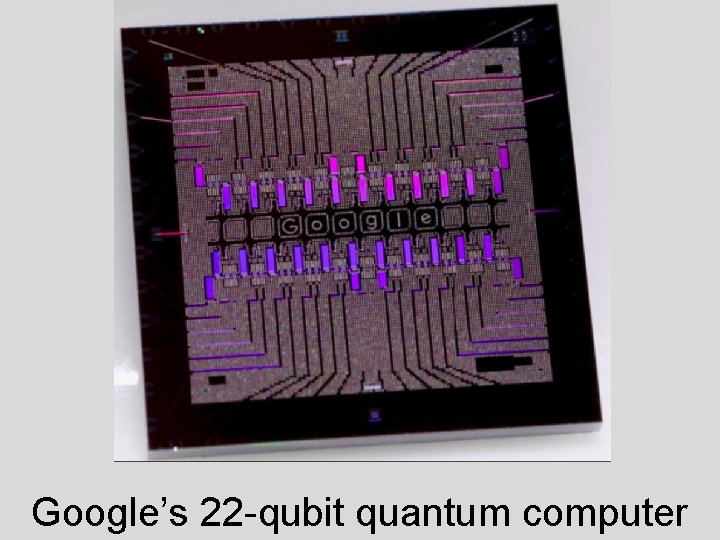
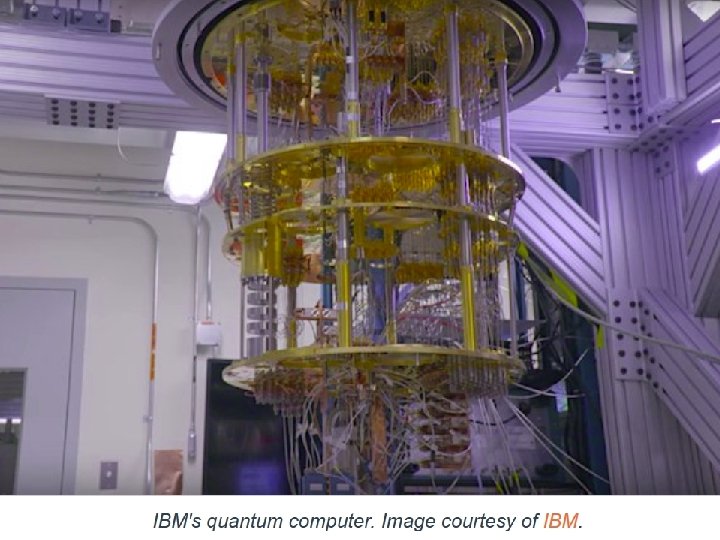
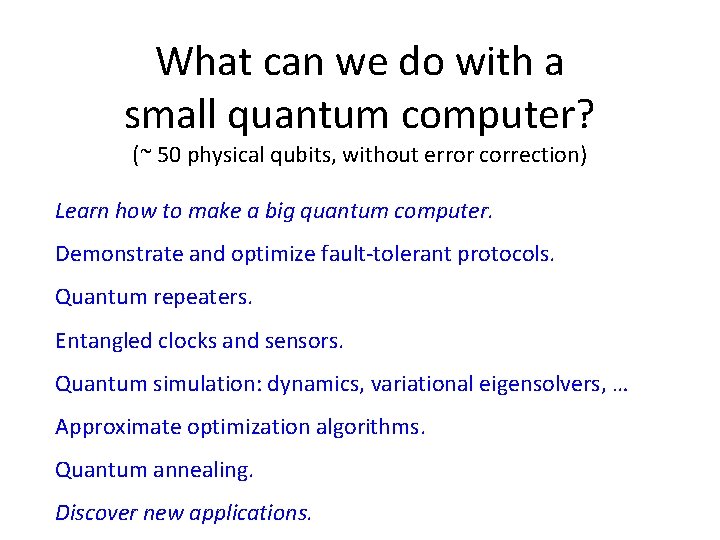
- Slides: 124
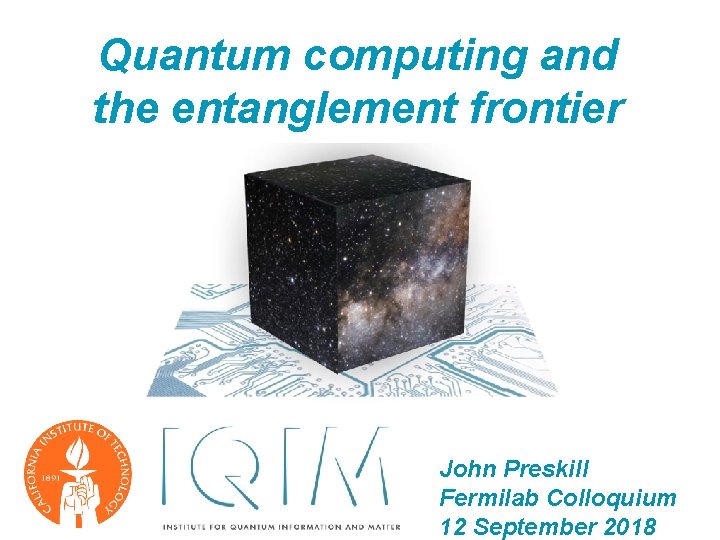
Quantum computing and the entanglement frontier John Preskill Fermilab Colloquium 12 September 2018
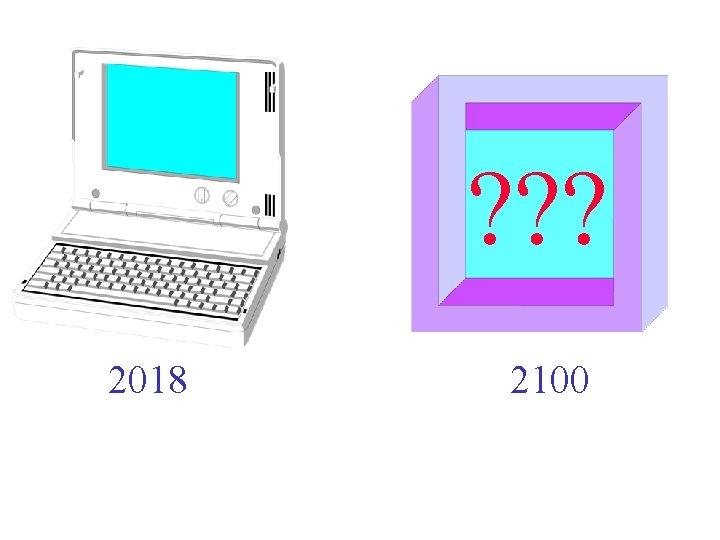
? ? ? 2018 2100
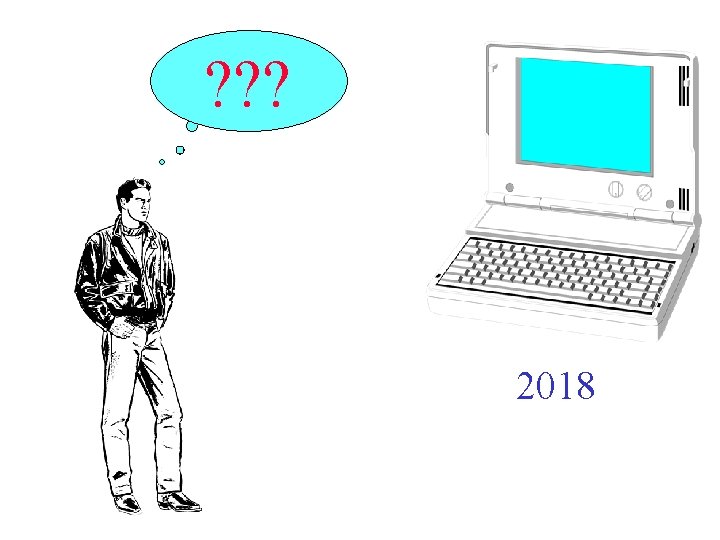
? ? ? 2018
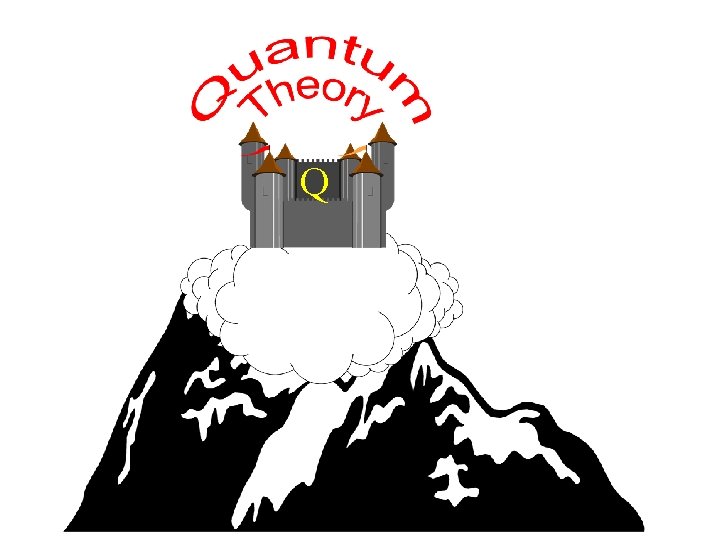
Q
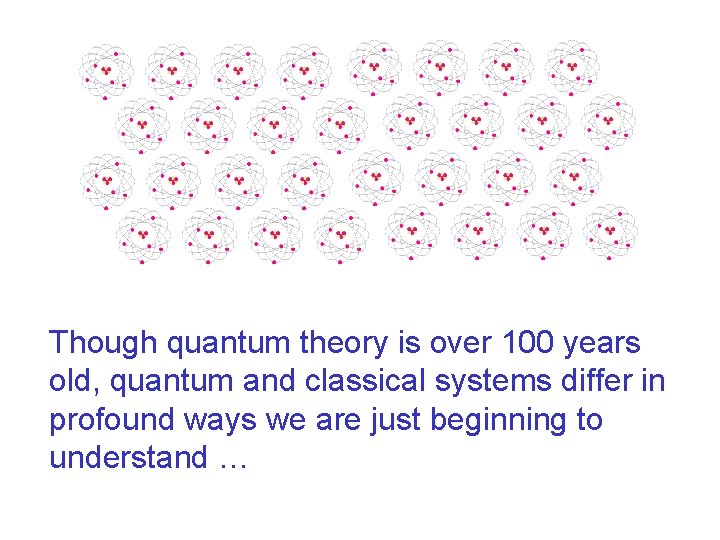
Though quantum theory is over 100 years old, quantum and classical systems differ in profound ways we are just beginning to understand …
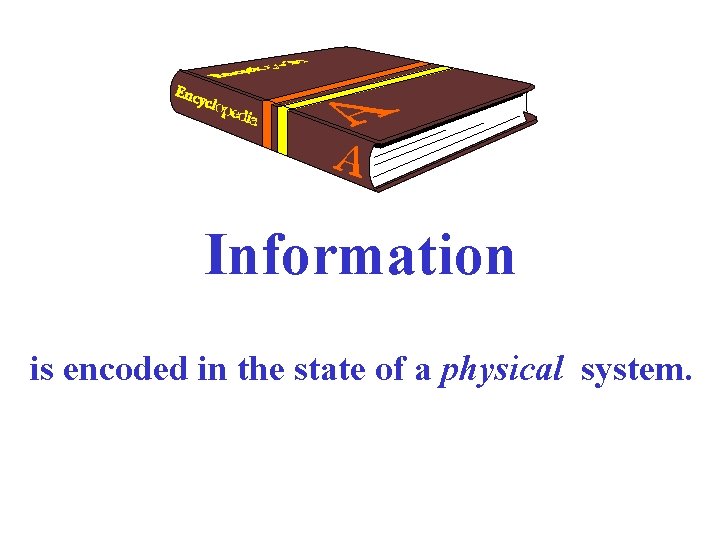
Information is encoded in the state of a physical system.
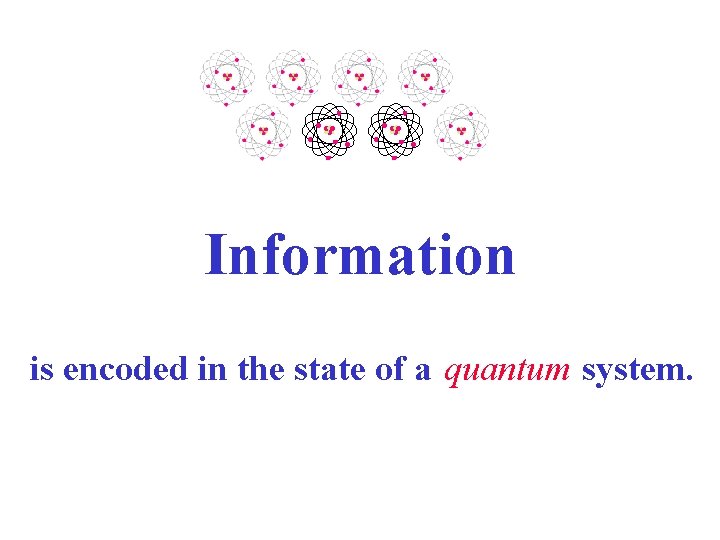
Information is encoded in the state of a quantum system.
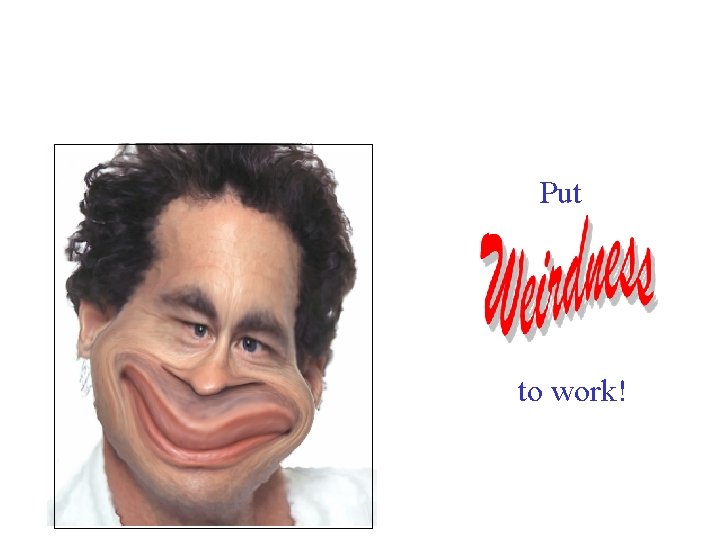
Put to work!
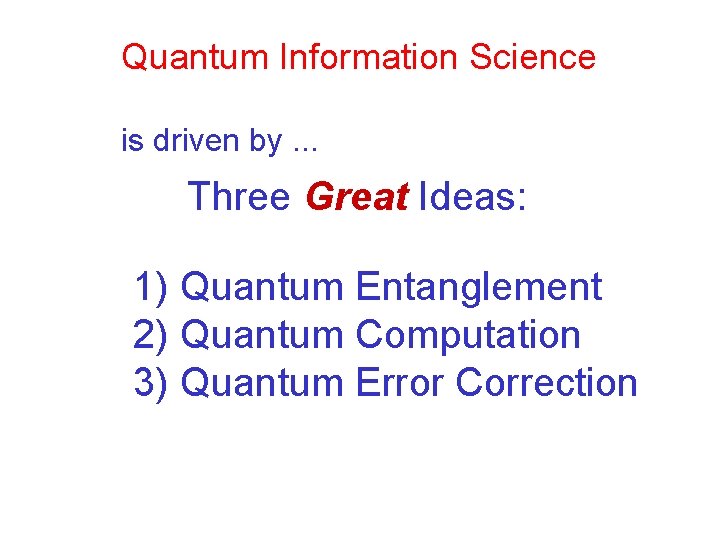
Quantum Information Science is driven by. . . Three Great Ideas: 1) Quantum Entanglement 2) Quantum Computation 3) Quantum Error Correction
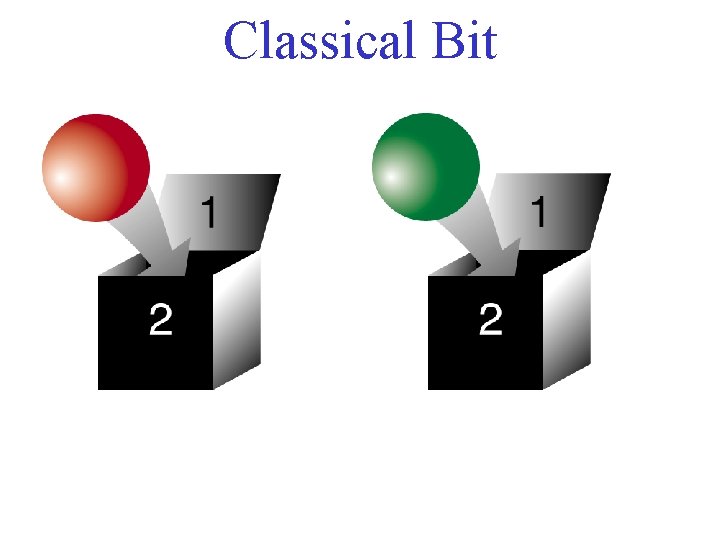
Classical Bit
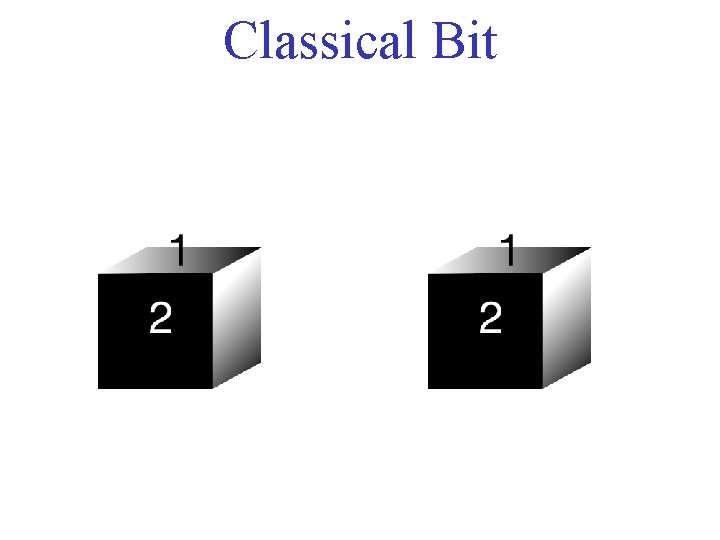
Classical Bit
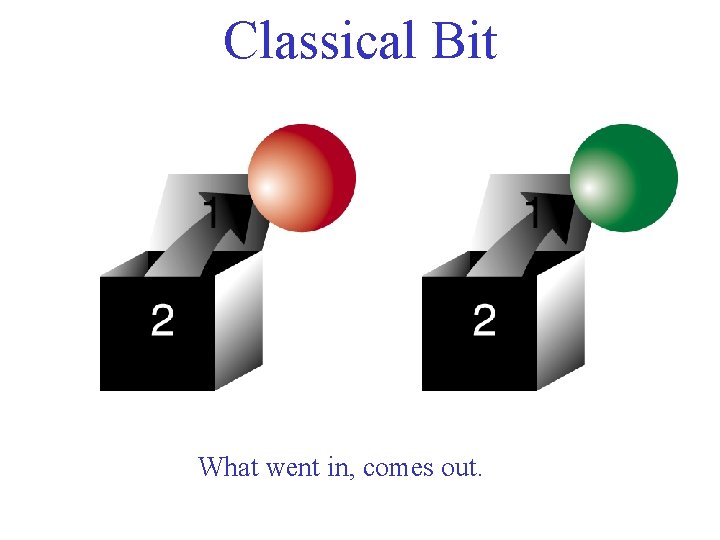
Classical Bit What went in, comes out.
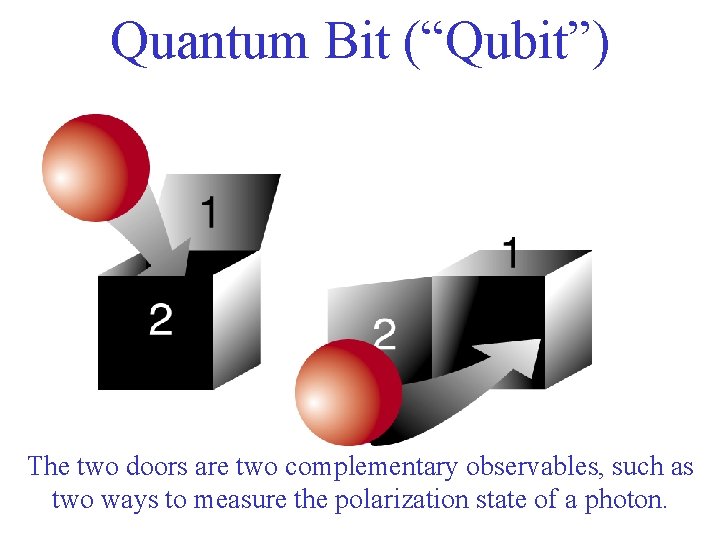
Quantum Bit (“Qubit”) The two doors are two complementary observables, such as two ways to measure the polarization state of a photon.
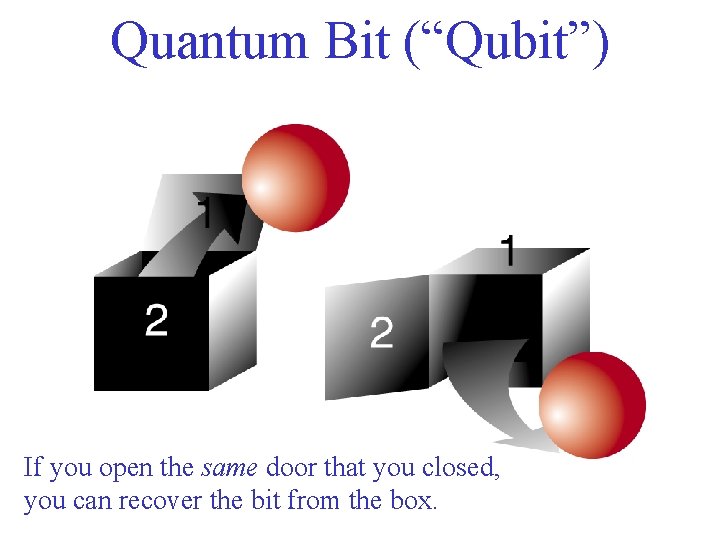
Quantum Bit (“Qubit”) If you open the same door that you closed, you can recover the bit from the box.
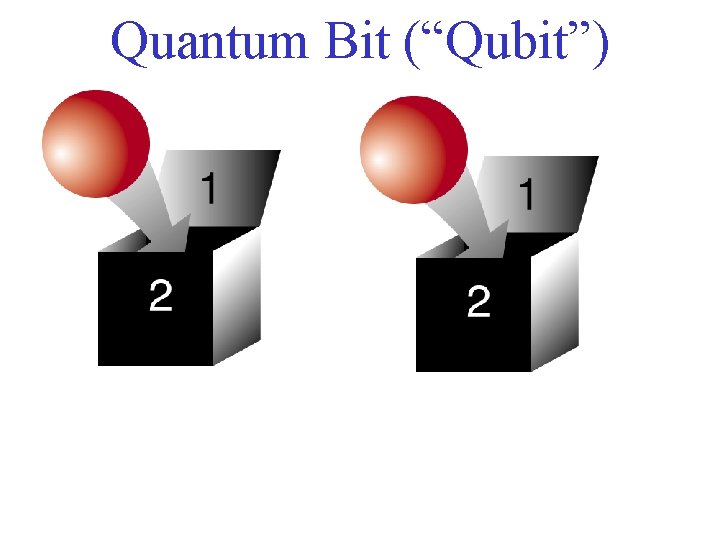
Quantum Bit (“Qubit”)
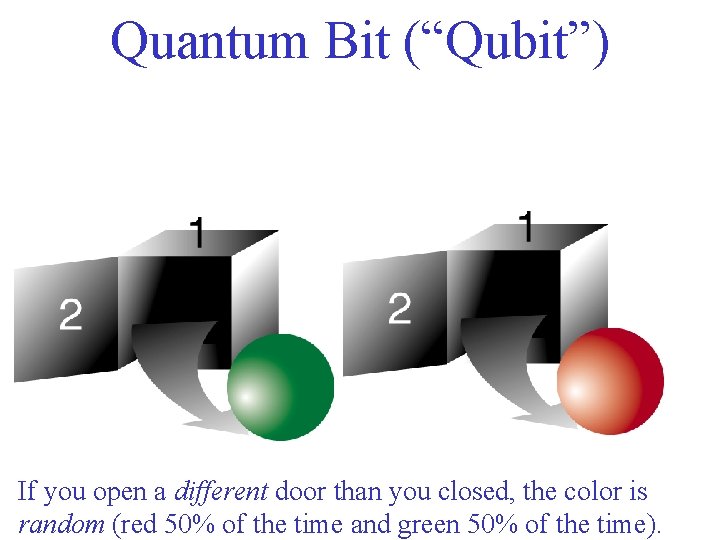
Quantum Bit (“Qubit”) If you open a different door than you closed, the color is random (red 50% of the time and green 50% of the time).
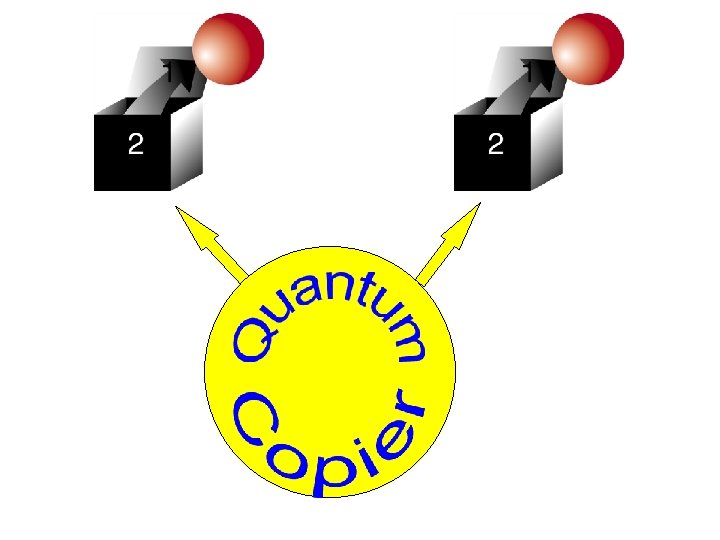
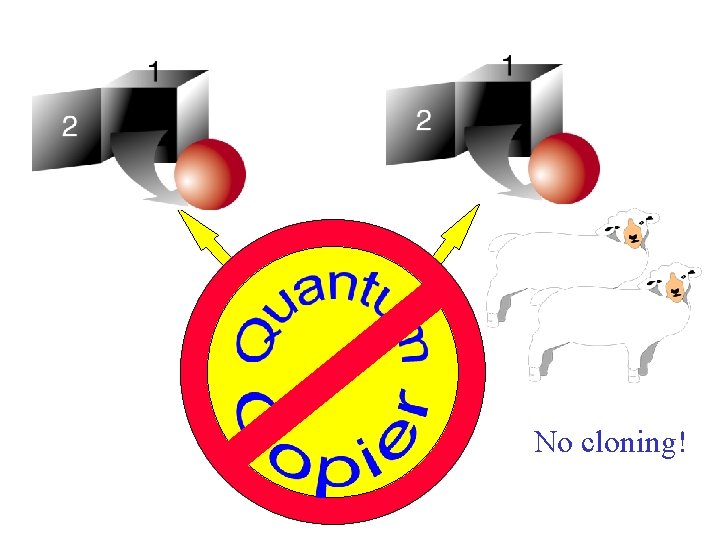
No cloning!
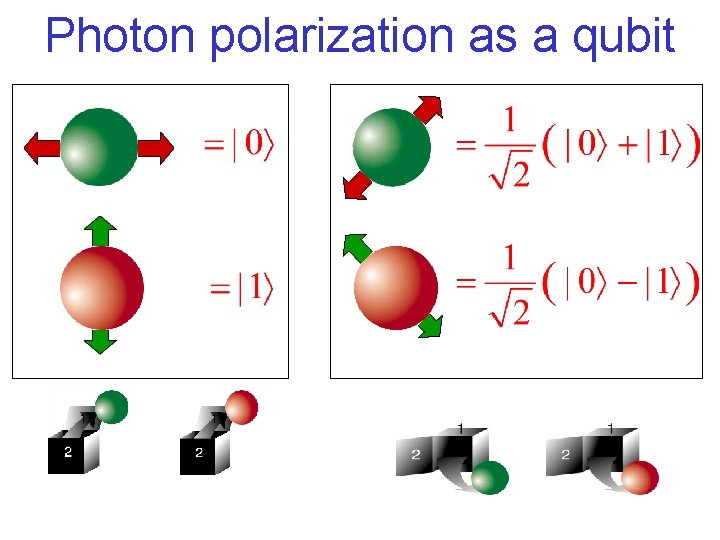
Photon polarization as a qubit
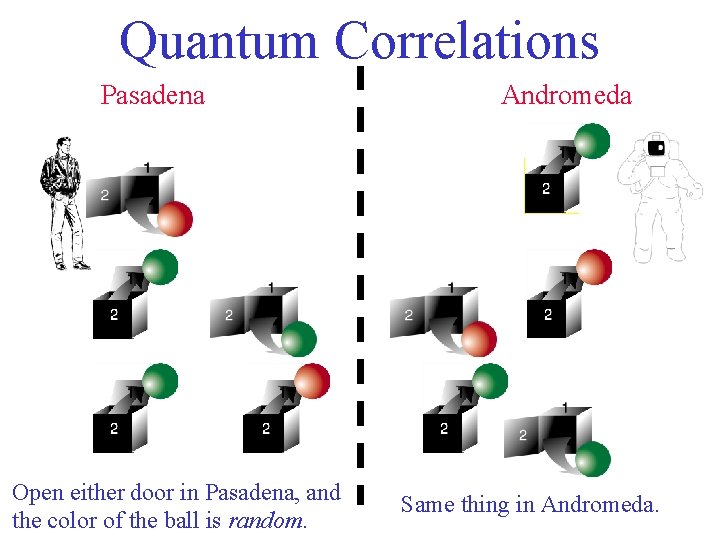
Quantum Correlations Pasadena Open either door in Pasadena, and the color of the ball is random. Andromeda Same thing in Andromeda.
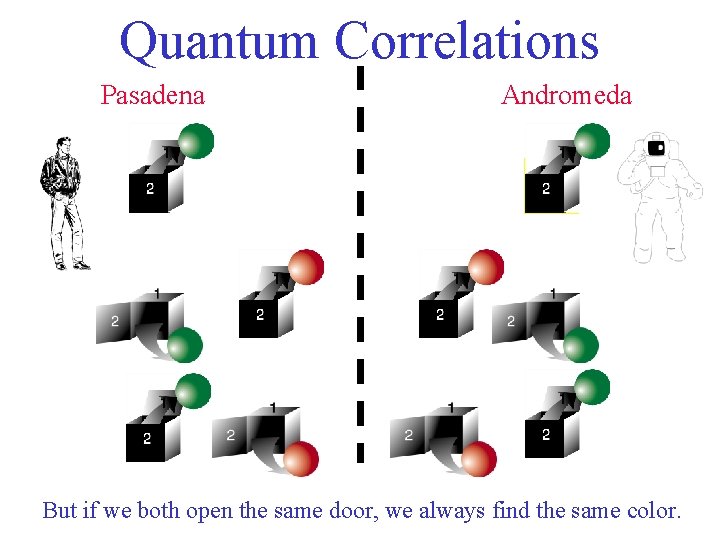
Quantum Correlations Pasadena Andromeda But if we both open the same door, we always find the same color.
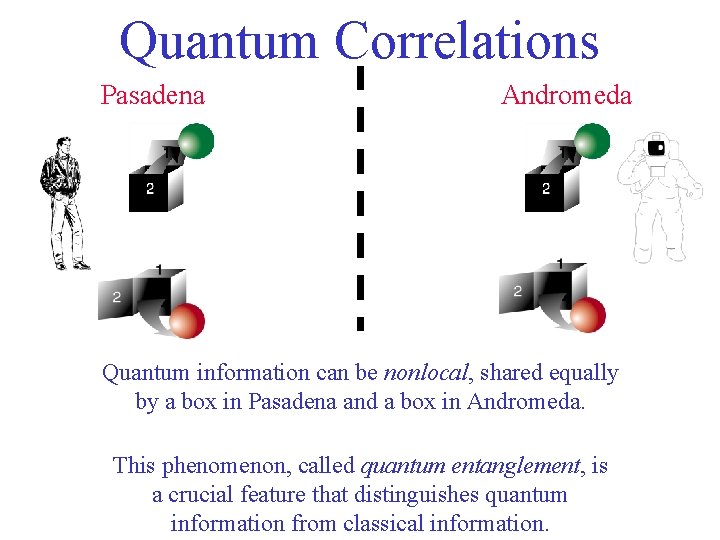
Quantum Correlations Pasadena Andromeda Quantum information can be nonlocal, shared equally by a box in Pasadena and a box in Andromeda. This phenomenon, called quantum entanglement, is a crucial feature that distinguishes quantum information from classical information.
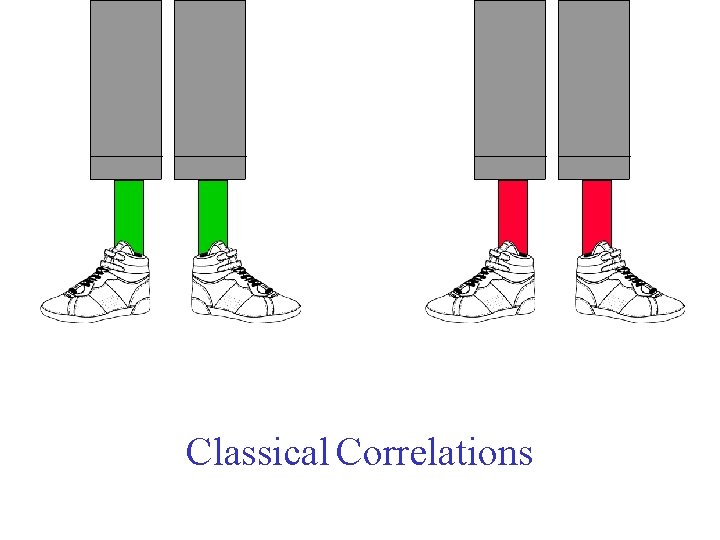
Classical Correlations
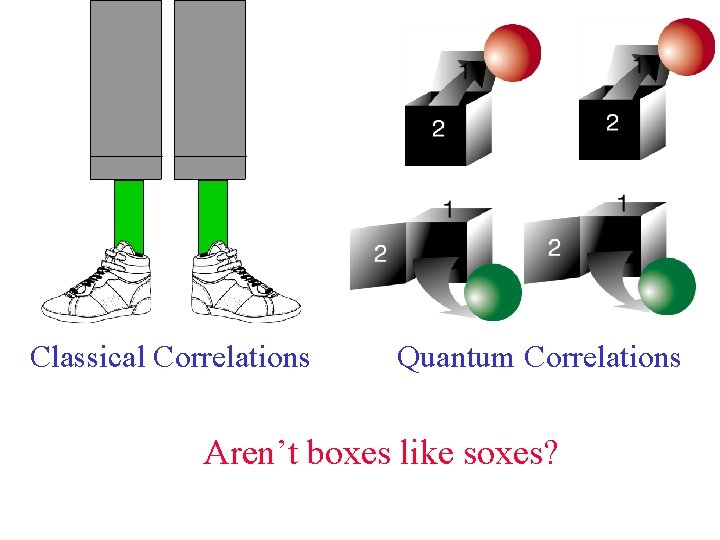
Classical Correlations Quantum Correlations Aren’t boxes like soxes?
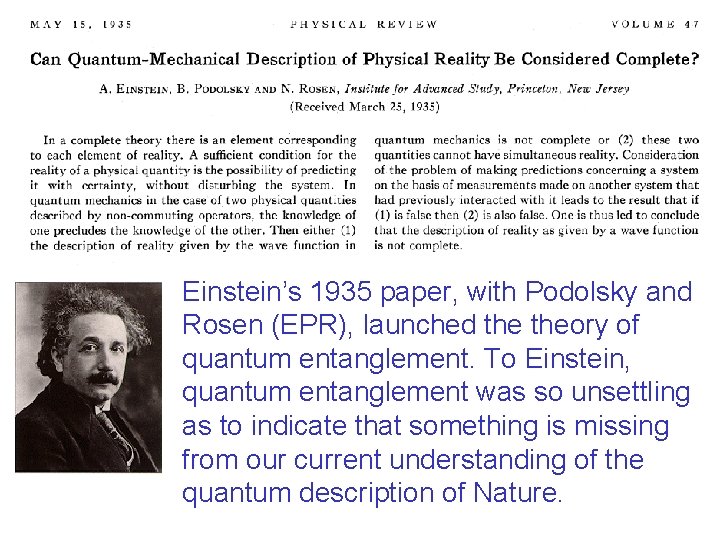
Einstein’s 1935 paper, with Podolsky and Rosen (EPR), launched theory of quantum entanglement. To Einstein, quantum entanglement was so unsettling as to indicate that something is missing from our current understanding of the quantum description of Nature.
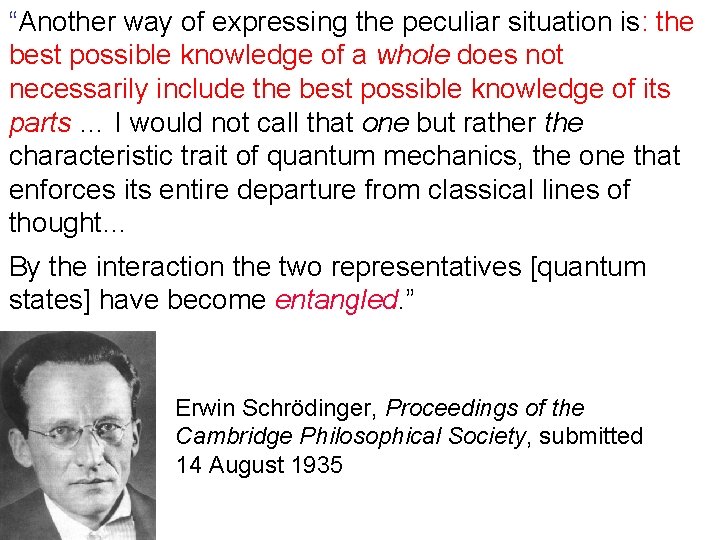
“Another way of expressing the peculiar situation is: the best possible knowledge of a whole does not necessarily include the best possible knowledge of its parts … I would not call that one but rather the characteristic trait of quantum mechanics, the one that enforces its entire departure from classical lines of thought… By the interaction the two representatives [quantum states] have become entangled. ” Erwin Schrödinger, Proceedings of the Cambridge Philosophical Society, submitted 14 August 1935
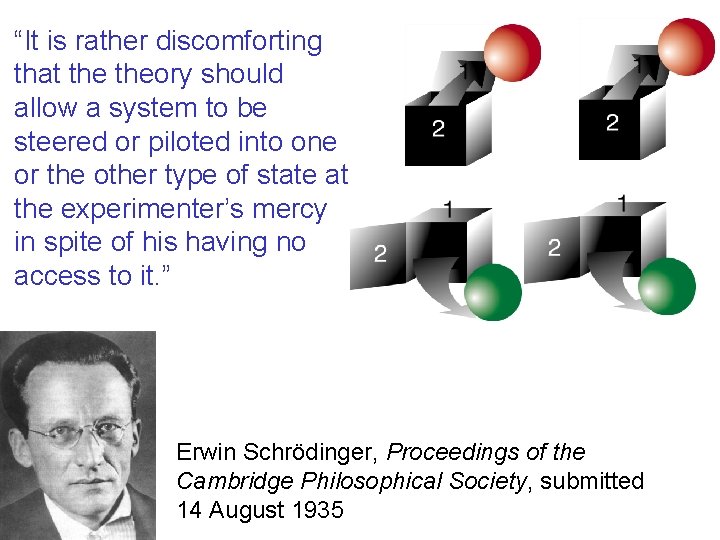
“It is rather discomforting that theory should allow a system to be steered or piloted into one or the other type of state at the experimenter’s mercy in spite of his having no access to it. ” Erwin Schrödinger, Proceedings of the Cambridge Philosophical Society, submitted 14 August 1935
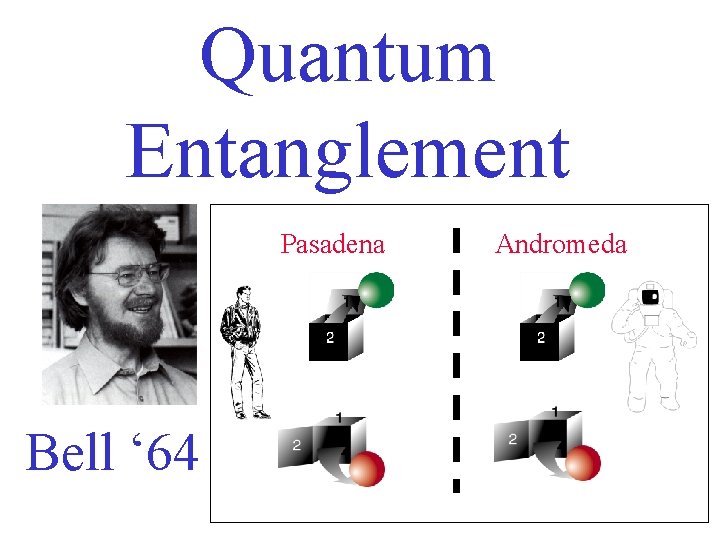
Quantum Entanglement Pasadena Bell ‘ 64 Andromeda
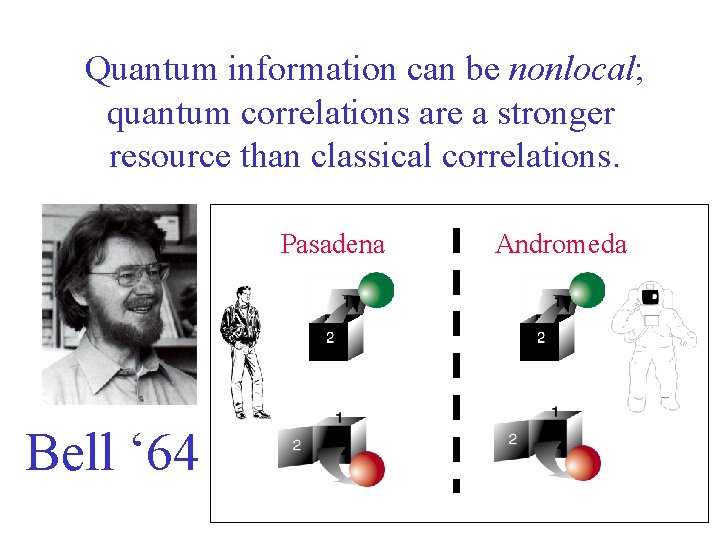
Quantum information can be nonlocal; quantum correlations are a stronger resource than classical correlations. Pasadena Bell ‘ 64 Andromeda
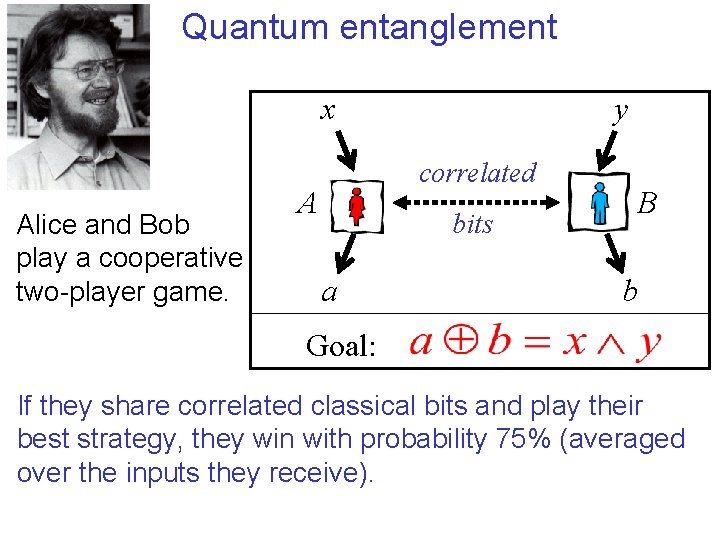
Quantum entanglement x Alice and Bob play a cooperative two-player game. y correlated A bits a B b Goal: If they share correlated classical bits and play their best strategy, they win with probability 75% (averaged over the inputs they receive).
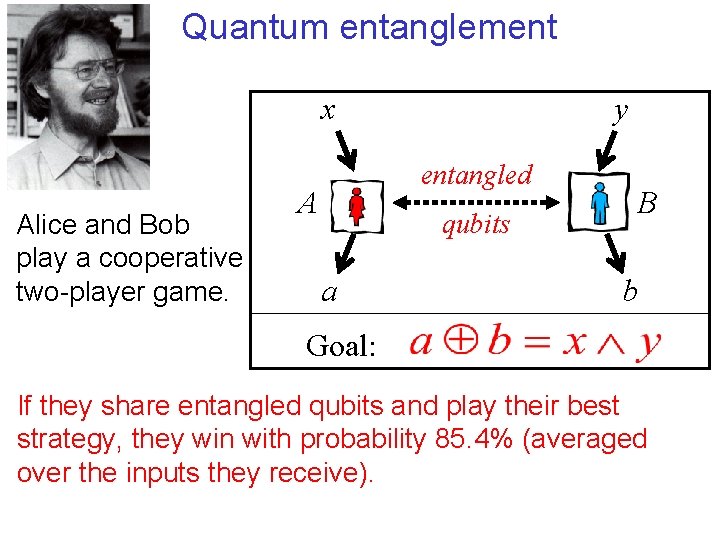
Quantum entanglement x Alice and Bob play a cooperative two-player game. y entangled A qubits a B b Goal: If they share entangled qubits and play their best strategy, they win with probability 85. 4% (averaged over the inputs they receive).
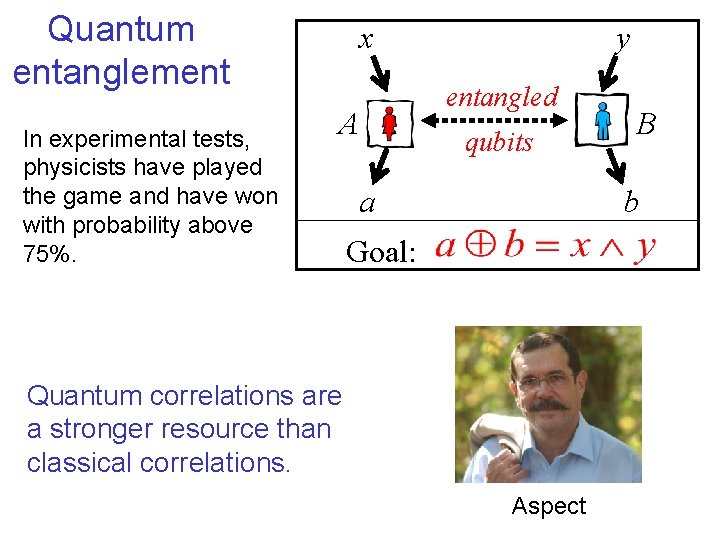
Quantum entanglement In experimental tests, physicists have played the game and have won with probability above 75%. x y entangled A qubits a B b Goal: Quantum correlations are a stronger resource than classical correlations. Aspect
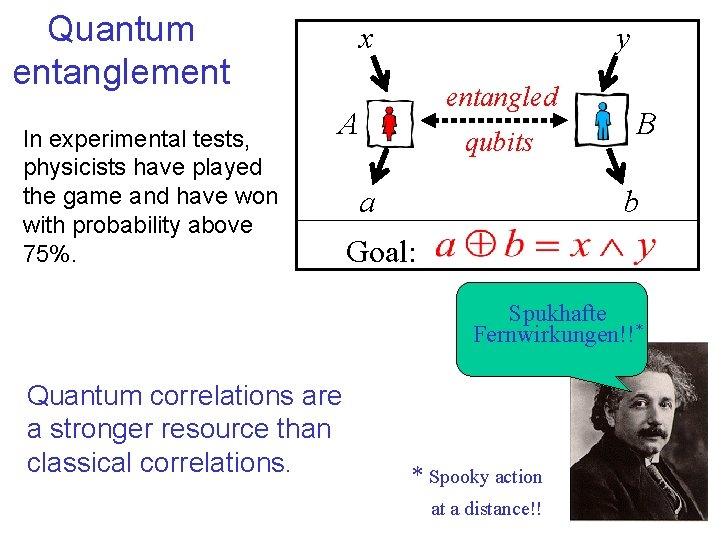
Quantum entanglement In experimental tests, physicists have played the game and have won with probability above 75%. x y entangled A qubits a B b Goal: Spukhafte Fernwirkungen!!* Quantum correlations are a stronger resource than classical correlations. * Spooky action at a distance!!
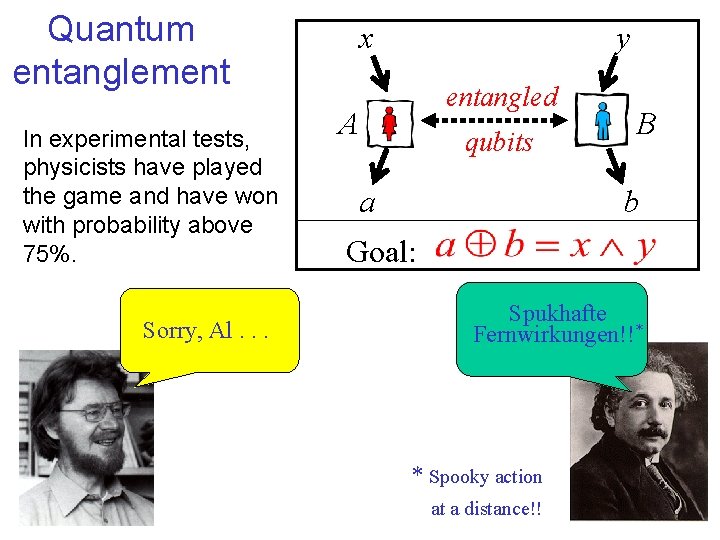
Quantum entanglement In experimental tests, physicists have played the game and have won with probability above 75%. Sorry, Al. . . x y entangled A qubits a B b Goal: Spukhafte Fernwirkungen!!* * Spooky action at a distance!!
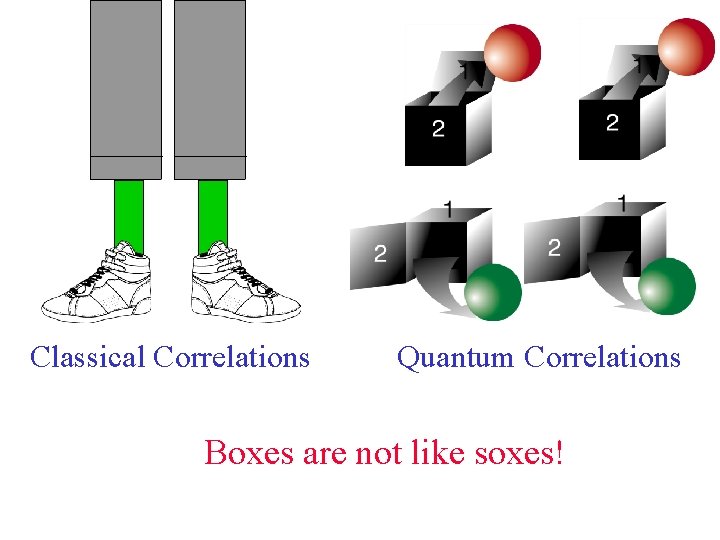
Classical Correlations Quantum Correlations Boxes are not like soxes!
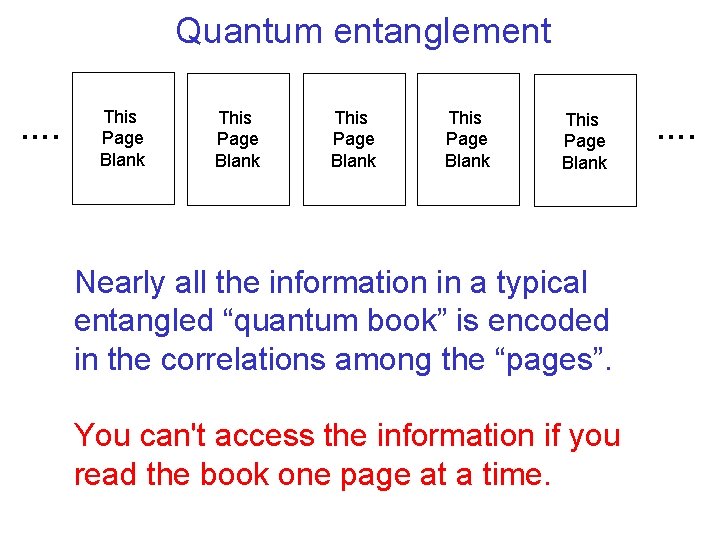
Quantum entanglement …. This Page Blank This Page Blank Nearly all the information in a typical entangled “quantum book” is encoded in the correlations among the “pages”. You can't access the information if you read the book one page at a time. ….
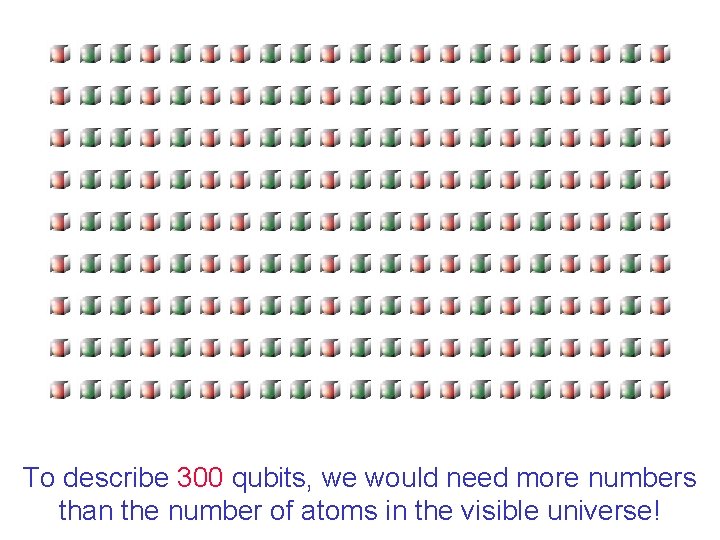
To describe 300 qubits, we would need more numbers than the number of atoms in the visible universe!
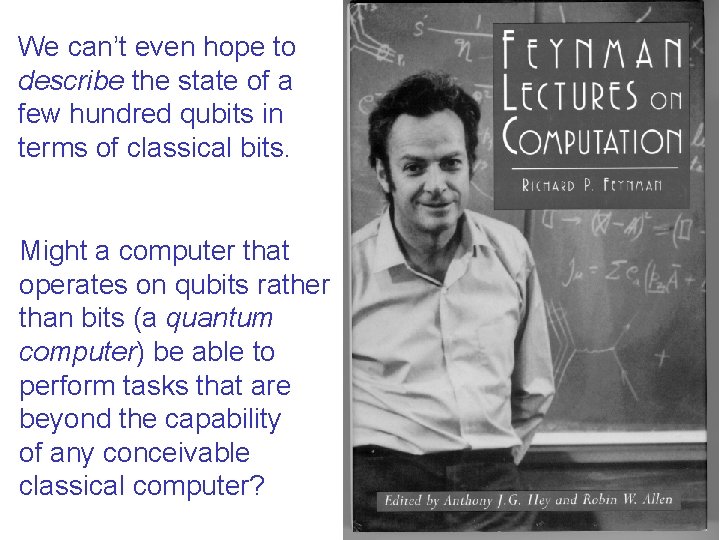
We can’t even hope to describe the state of a few hundred qubits in terms of classical bits. Might a computer that operates on qubits rather than bits (a quantum computer) be able to perform tasks that are beyond the capability of any conceivable classical computer?
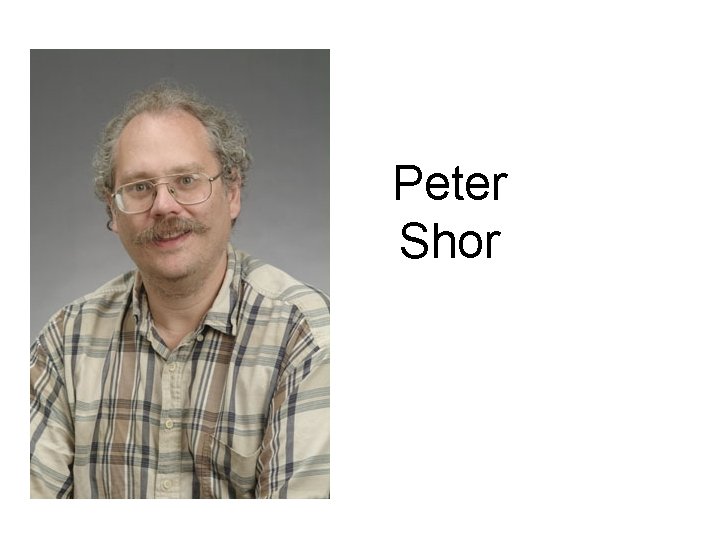
Peter Shor
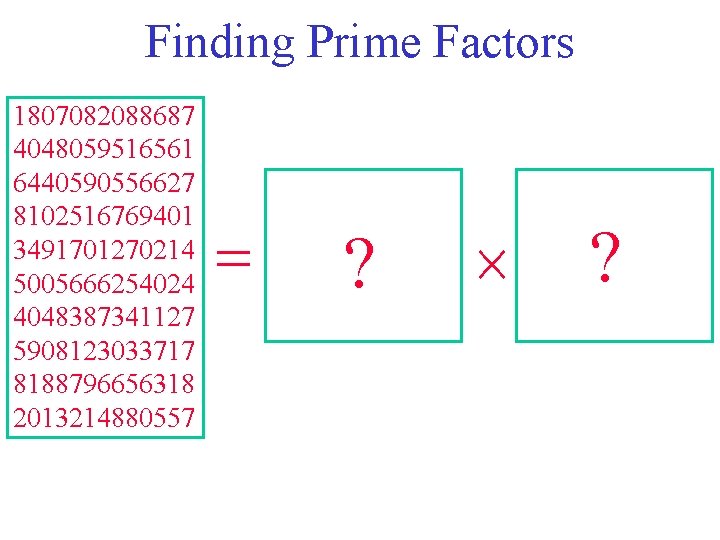
Finding Prime Factors 1807082088687 4048059516561 6440590556627 8102516769401 3491701270214 5005666254024 4048387341127 5908123033717 8188796656318 2013214880557 = ? ´ ?
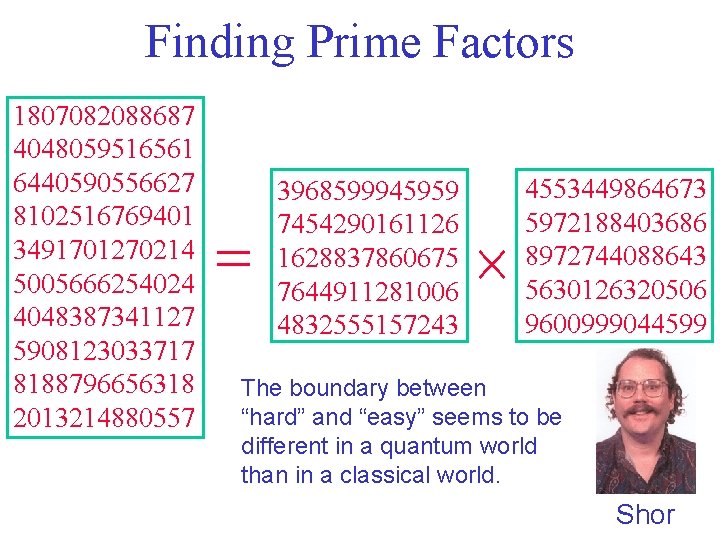
Finding Prime Factors 1807082088687 4048059516561 6440590556627 8102516769401 3491701270214 5005666254024 4048387341127 5908123033717 8188796656318 2013214880557 = 3968599945959 7454290161126 1628837860675 7644911281006 4832555157243 ´ 4553449864673 5972188403686 8972744088643 5630126320506 9600999044599 The boundary between “hard” and “easy” seems to be different in a quantum world than in a classical world. Shor
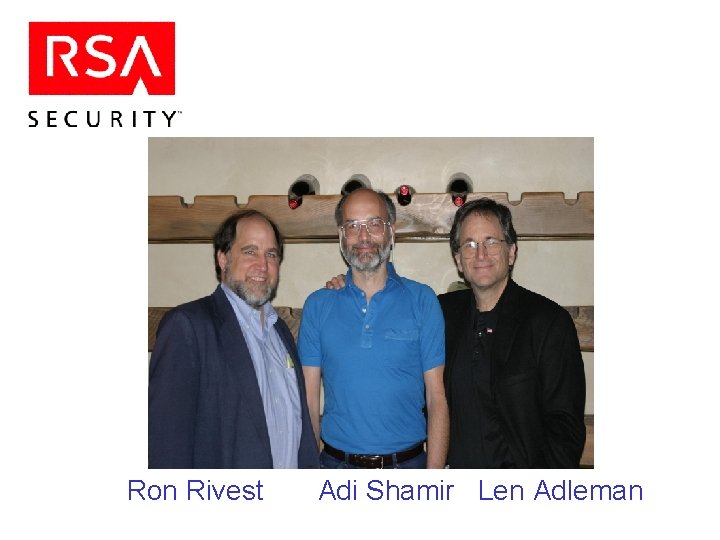
Ron Rivest Adi Shamir Len Adleman
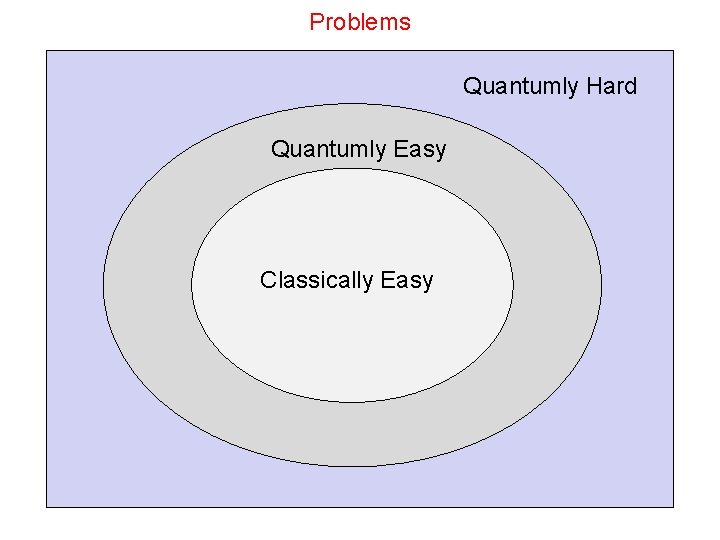
Problems Quantumly Hard Quantumly Easy Classically Easy
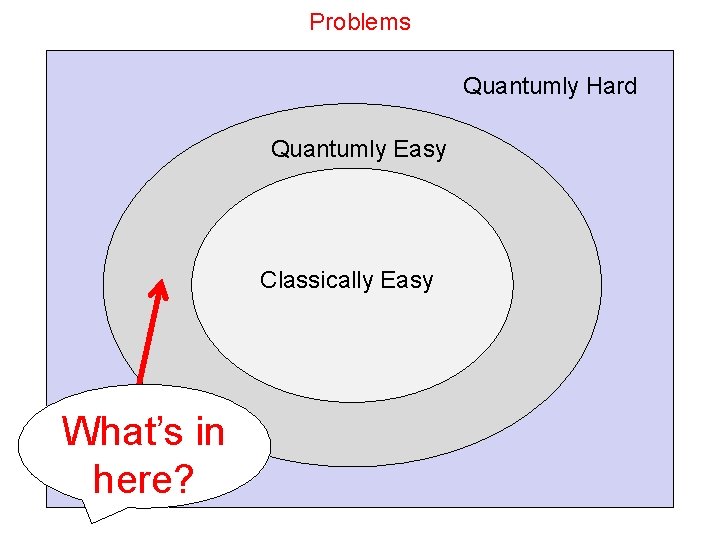
Problems Quantumly Hard Quantumly Easy Classically Easy What’s in here?
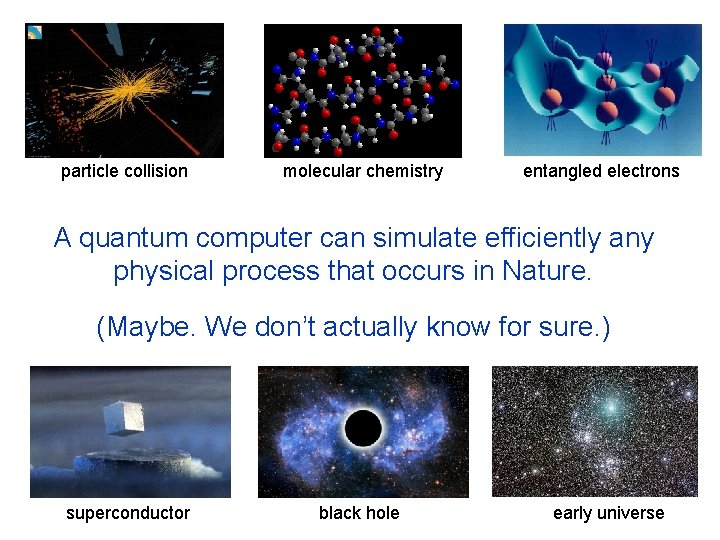
particle collision molecular chemistry entangled electrons A quantum computer can simulate efficiently any physical process that occurs in Nature. (Maybe. We don’t actually know for sure. ) superconductor black hole early universe
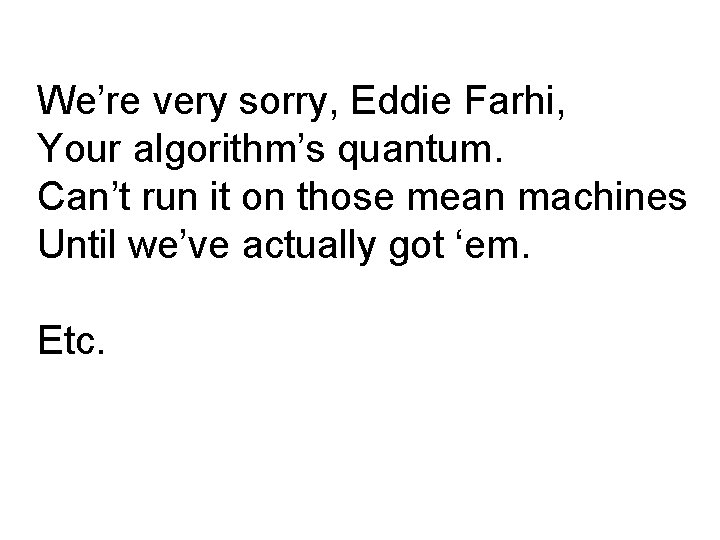
We’re very sorry, Eddie Farhi, Your algorithm’s quantum. Can’t run it on those mean machines Until we’ve actually got ‘em. Etc.
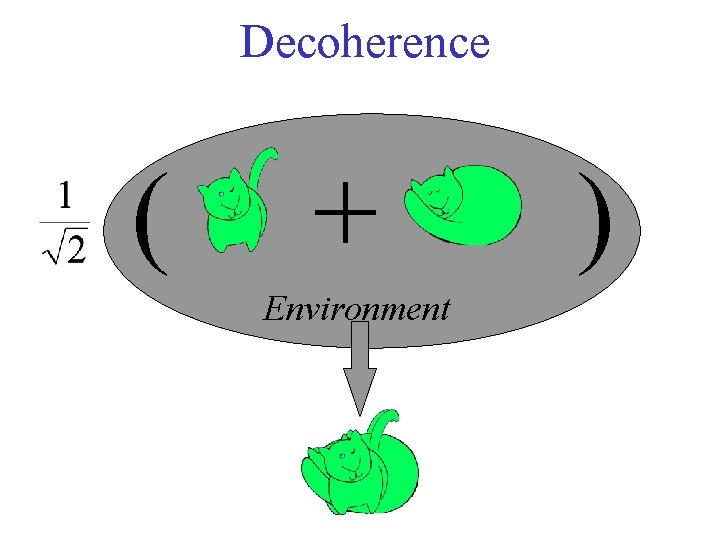
Decoherence ( + Environment )
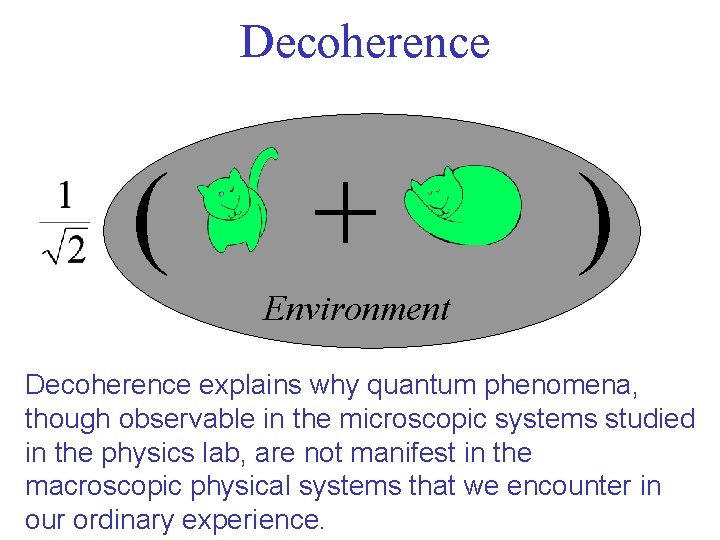
Decoherence ( + ) Environment Decoherence explains why quantum phenomena, though observable in the microscopic systems studied in the physics lab, are not manifest in the macroscopic physical systems that we encounter in our ordinary experience.
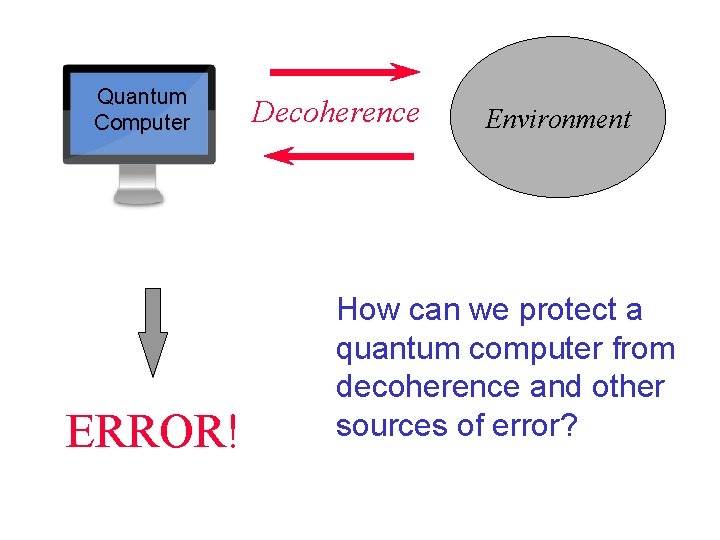
Quantum Computer ERROR! Decoherence Environment How can we protect a quantum computer from decoherence and other sources of error?
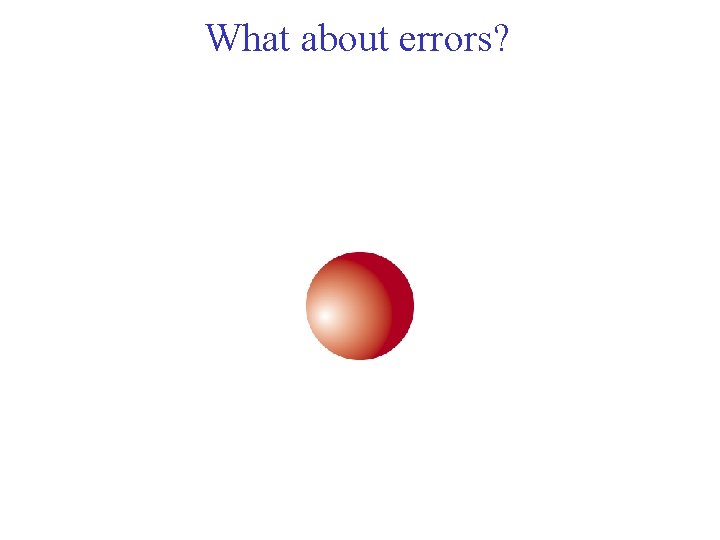
What about errors?
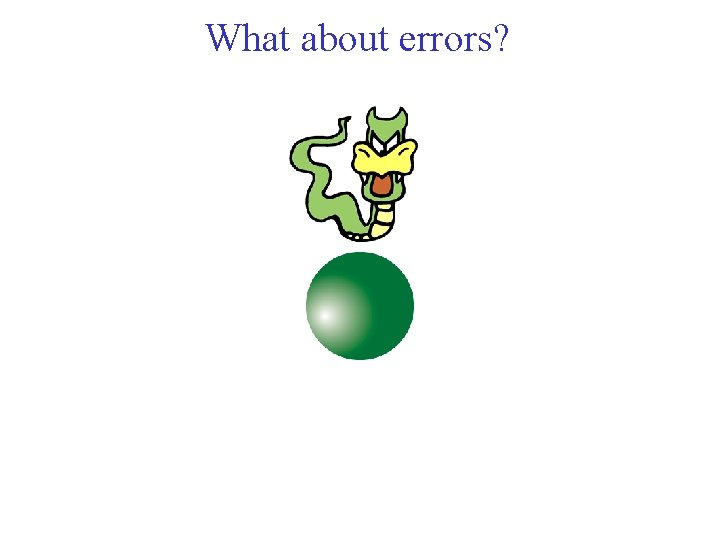
What about errors?
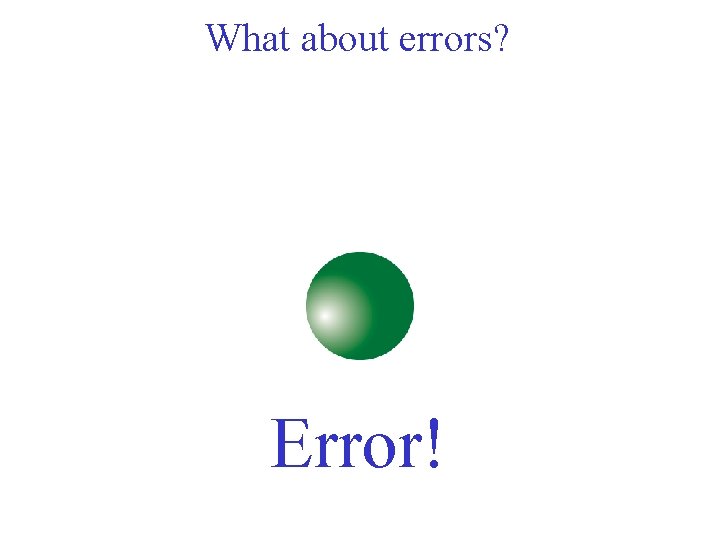
What about errors? Error!
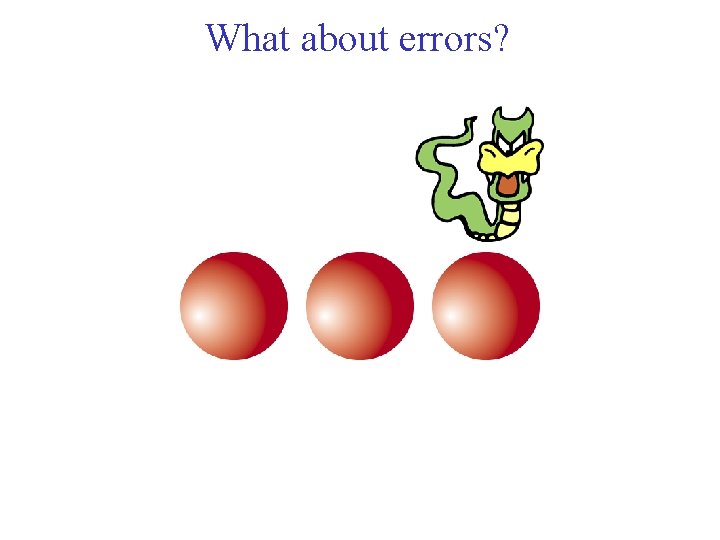
What about errors?
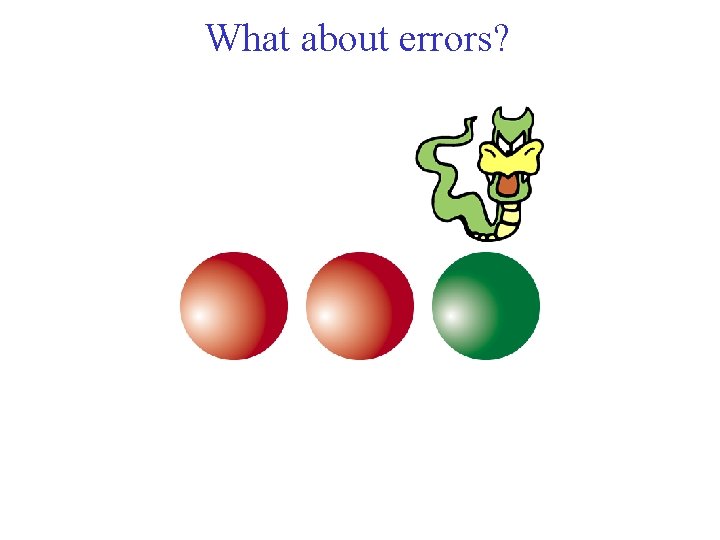
What about errors?
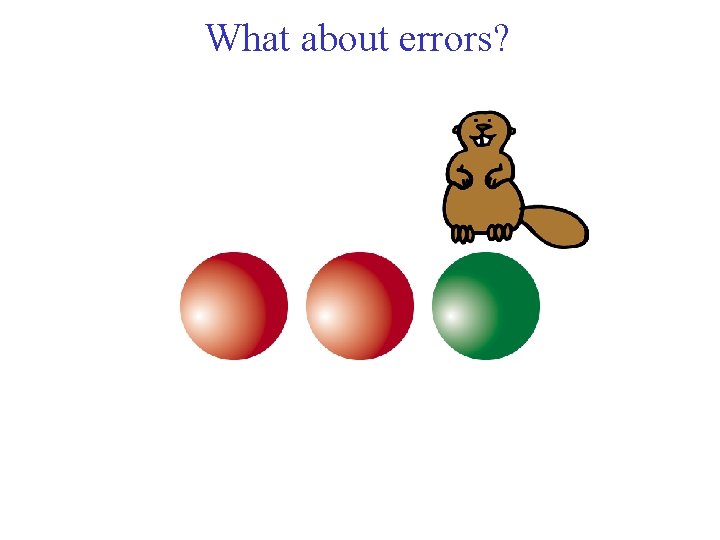
What about errors?
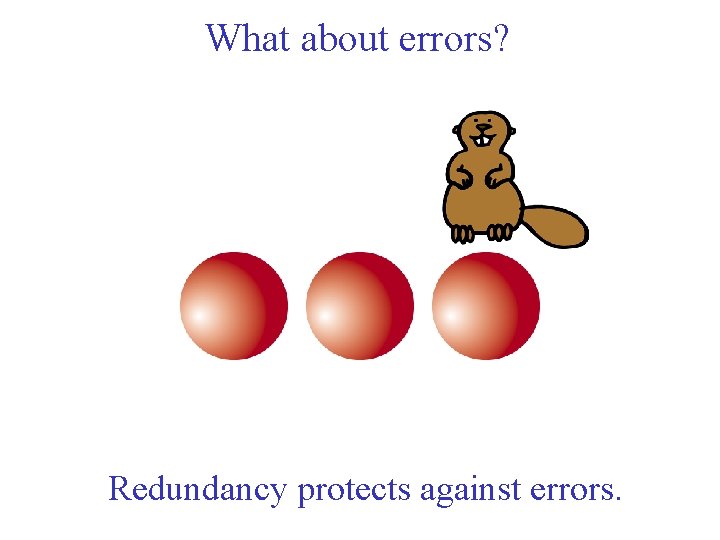
What about errors? Redundancy protects against errors.
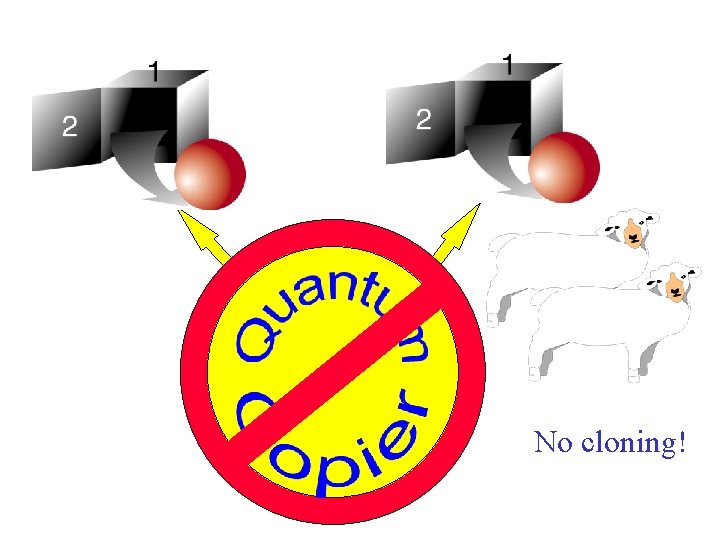
No cloning!
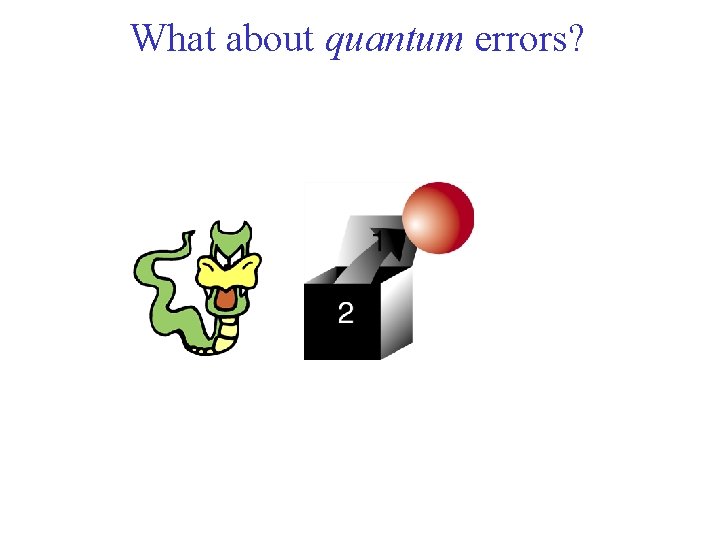
What about quantum errors?
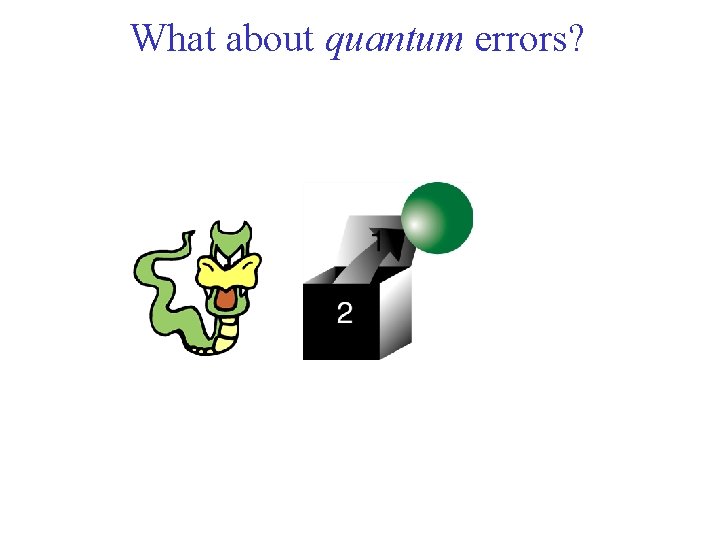
What about quantum errors?
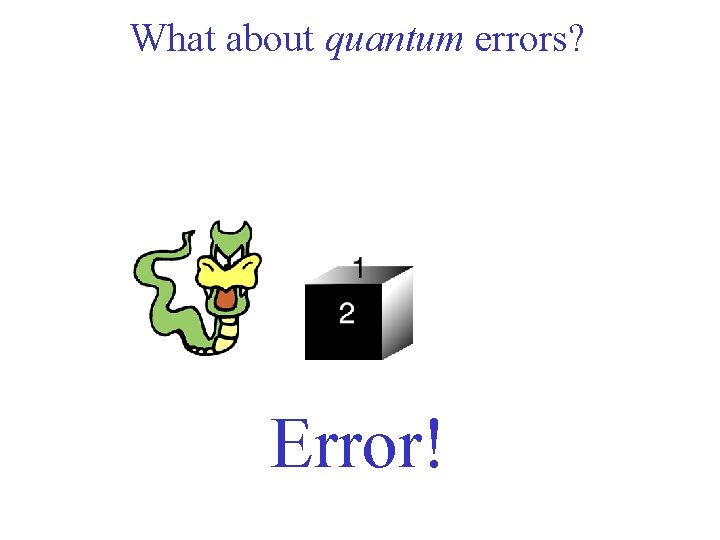
What about quantum errors? Error!
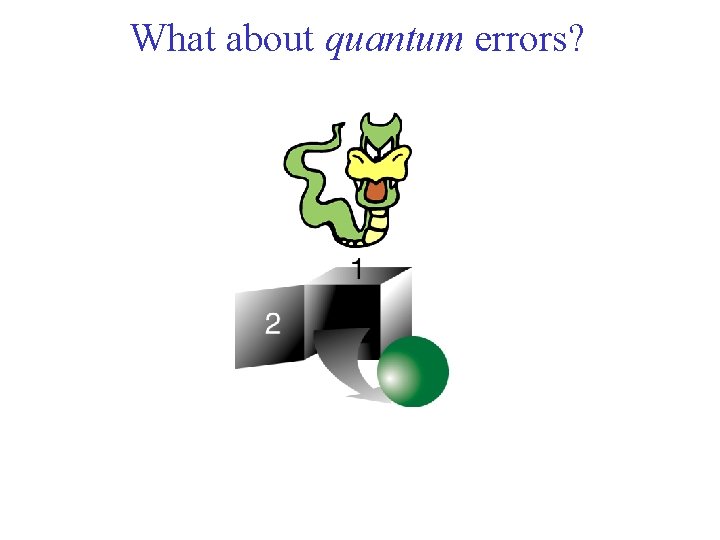
What about quantum errors?
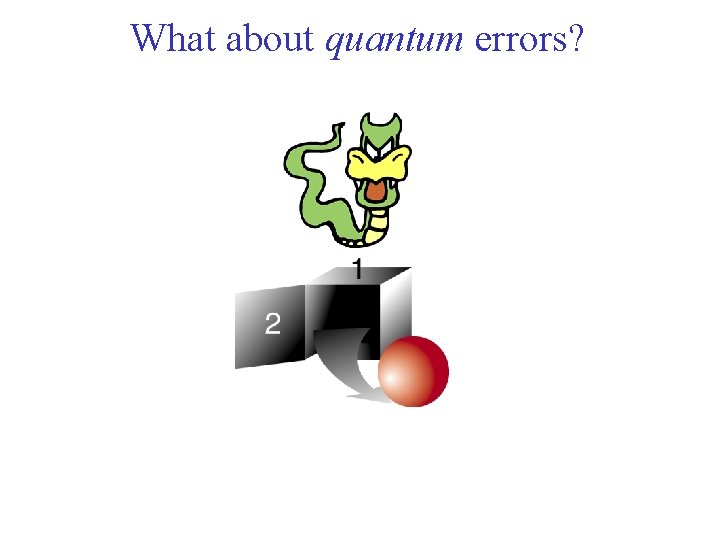
What about quantum errors?
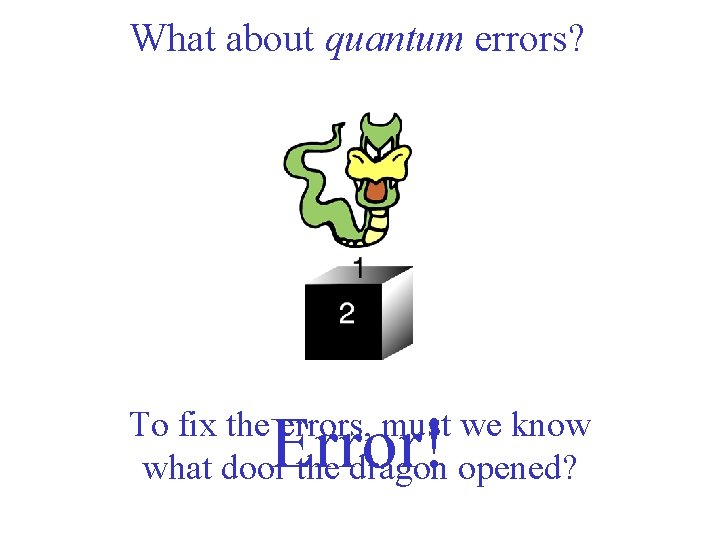
What about quantum errors? Error! To fix the errors, must we know what door the dragon opened?
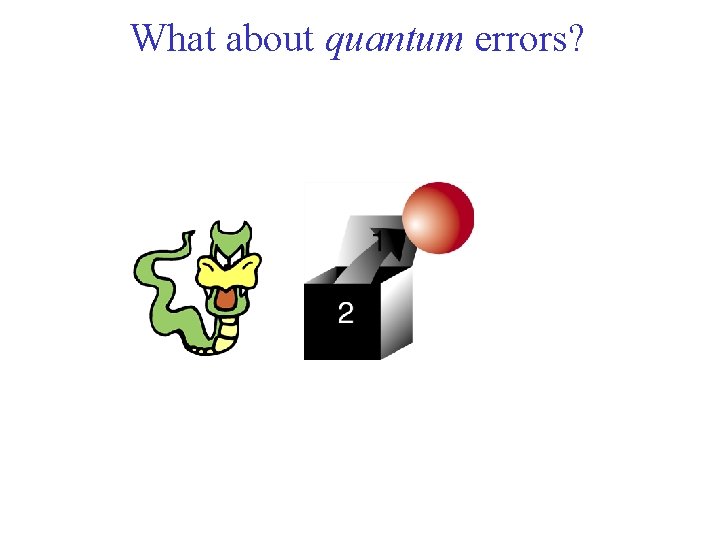
What about quantum errors?
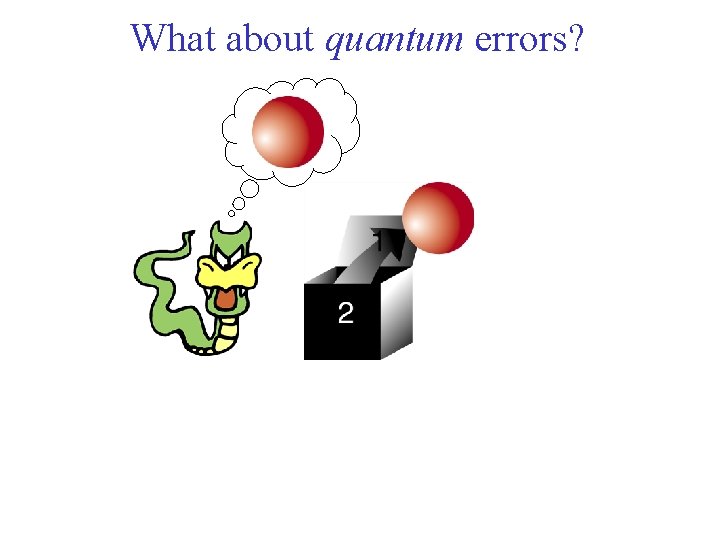
What about quantum errors?
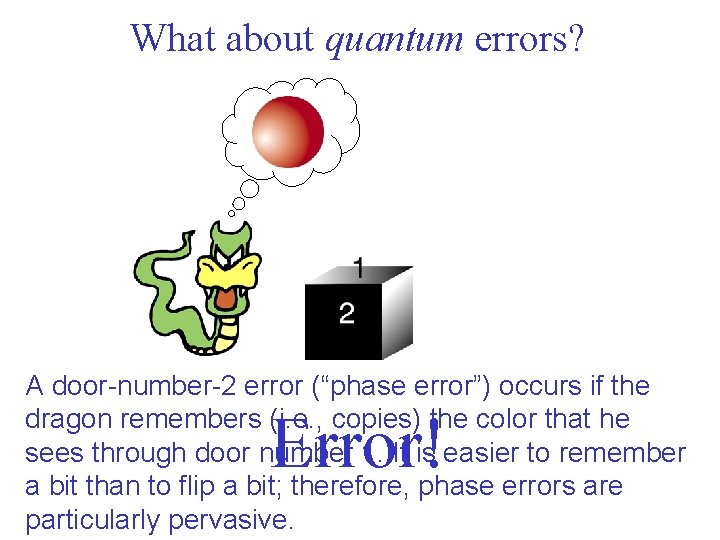
What about quantum errors? A door-number-2 error (“phase error”) occurs if the dragon remembers (i. e. , copies) the color that he sees through door number 1. It is easier to remember a bit than to flip a bit; therefore, phase errors are particularly pervasive. Error!
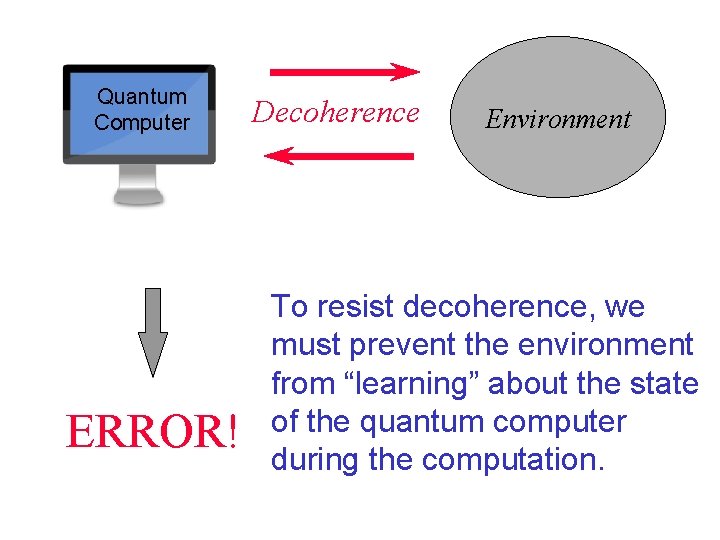
Quantum Computer ERROR! Decoherence Environment To resist decoherence, we must prevent the environment from “learning” about the state of the quantum computer during the computation.
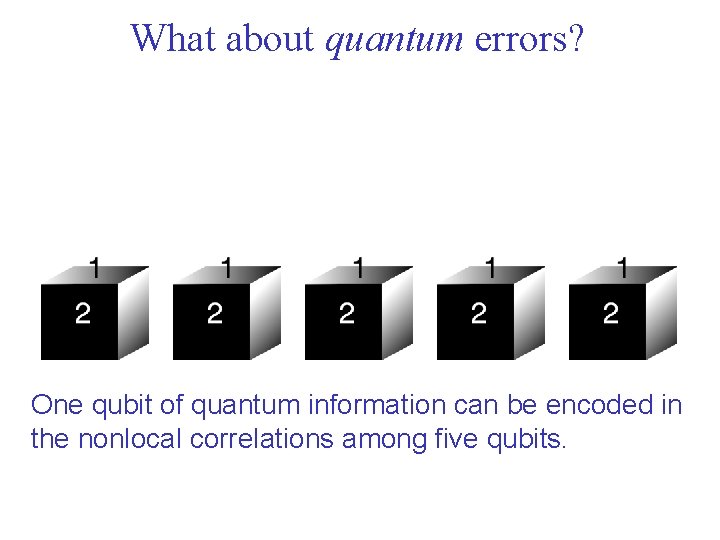
What about quantum errors? One qubit of quantum information can be encoded in the nonlocal correlations among five qubits.
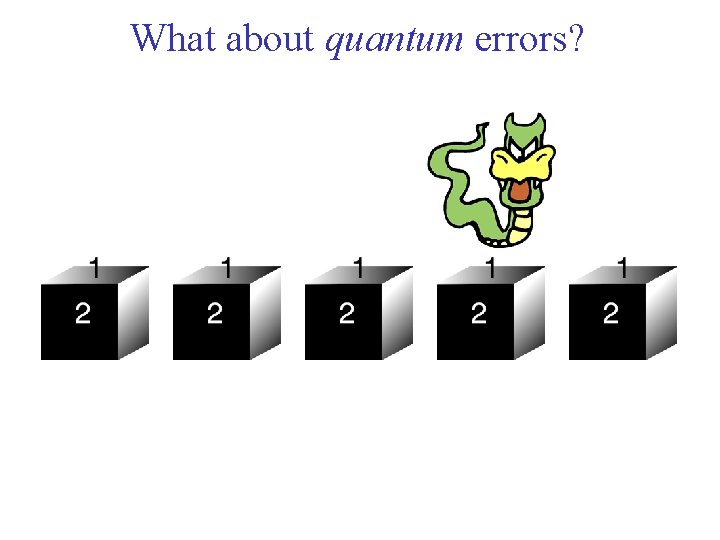
What about quantum errors?
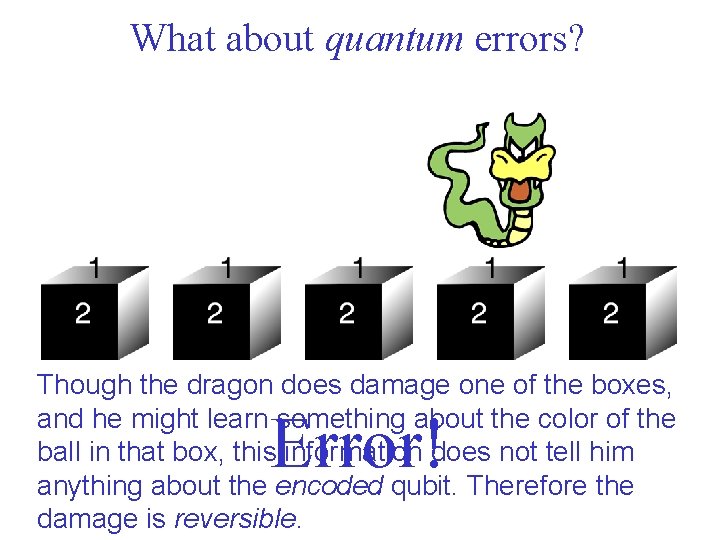
What about quantum errors? Though the dragon does damage one of the boxes, and he might learn something about the color of the ball in that box, this information does not tell him anything about the encoded qubit. Therefore the damage is reversible. Error!
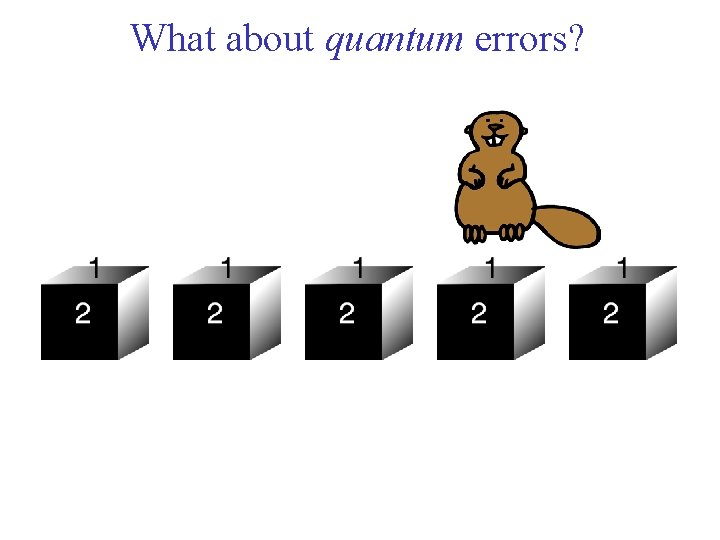
What about quantum errors?
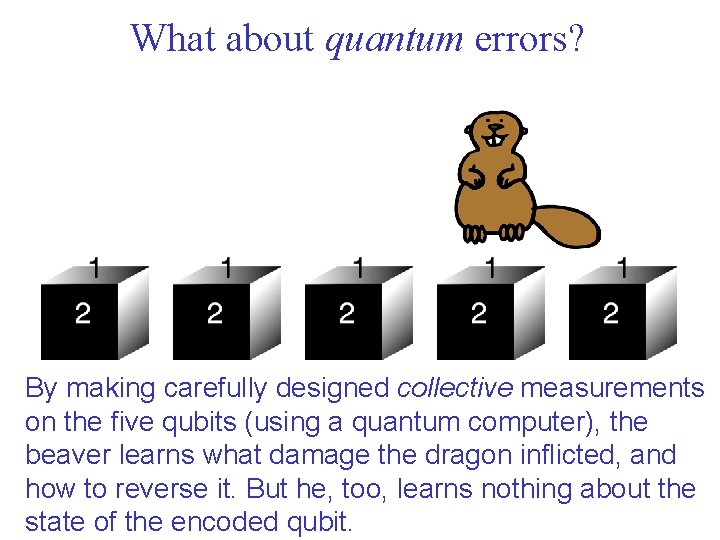
What about quantum errors? By making carefully designed collective measurements on the five qubits (using a quantum computer), the beaver learns what damage the dragon inflicted, and how to reverse it. But he, too, learns nothing about the state of the encoded qubit.
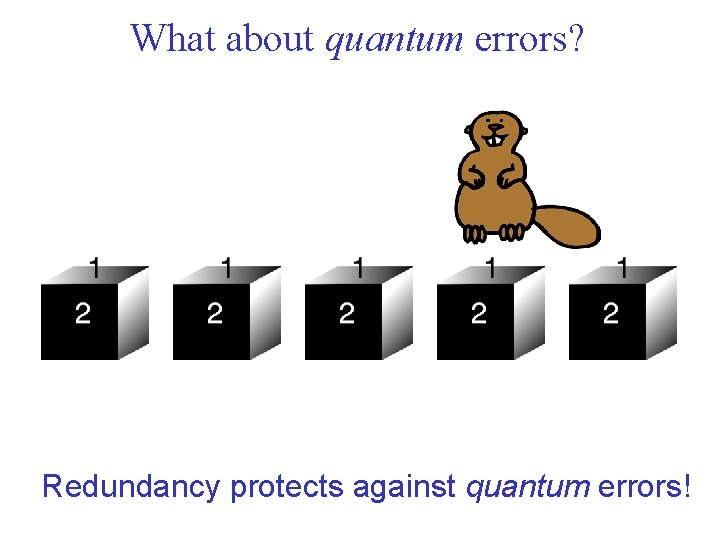
What about quantum errors? Redundancy protects against quantum errors!
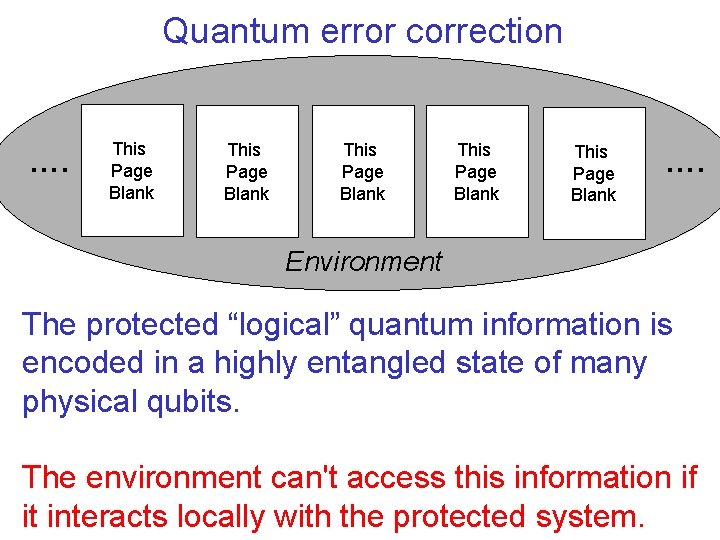
Quantum error correction …. This Page Blank This Page Blank …. Environment The protected “logical” quantum information is encoded in a highly entangled state of many physical qubits. The environment can't access this information if it interacts locally with the protected system.
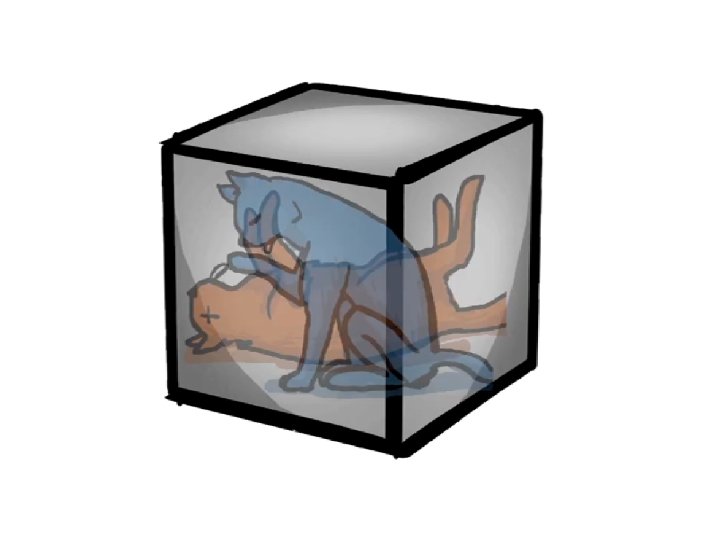
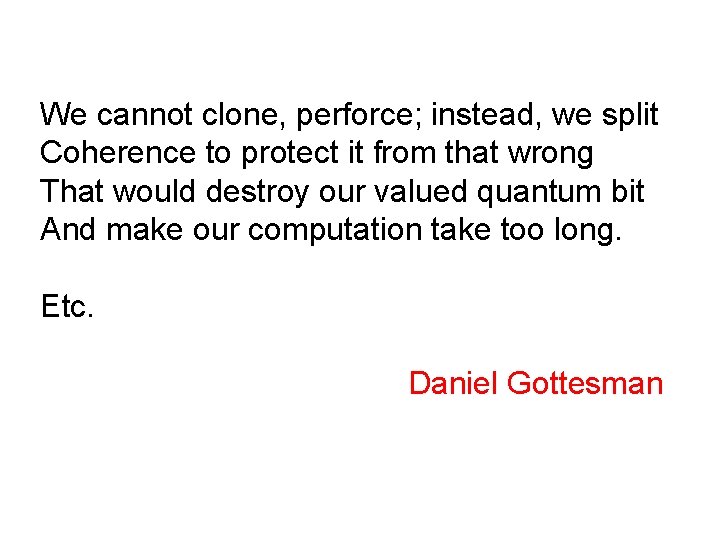
We cannot clone, perforce; instead, we split Coherence to protect it from that wrong That would destroy our valued quantum bit And make our computation take too long. Etc. Daniel Gottesman
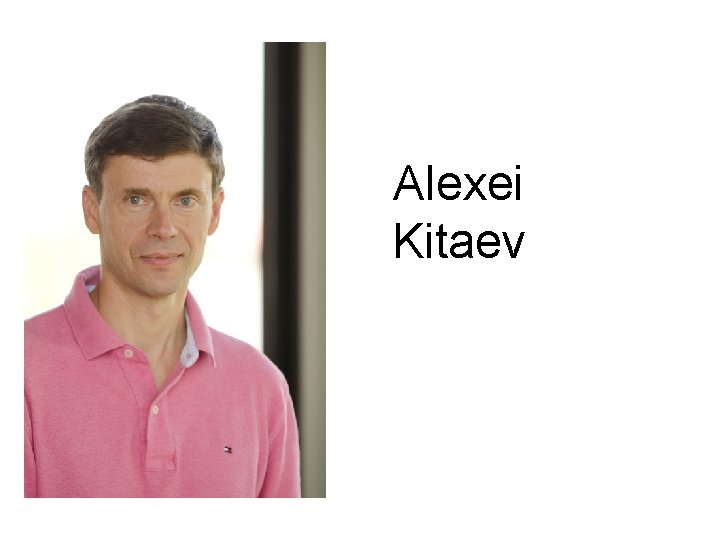
Alexei Kitaev
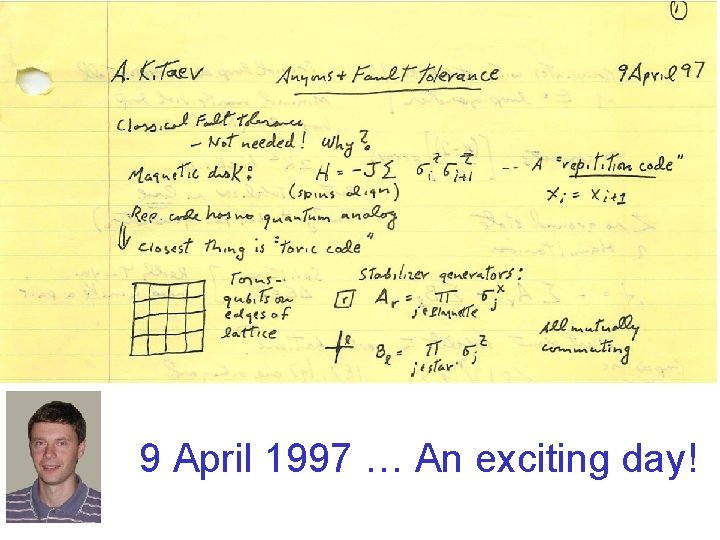
9 April 1997 … An exciting day!
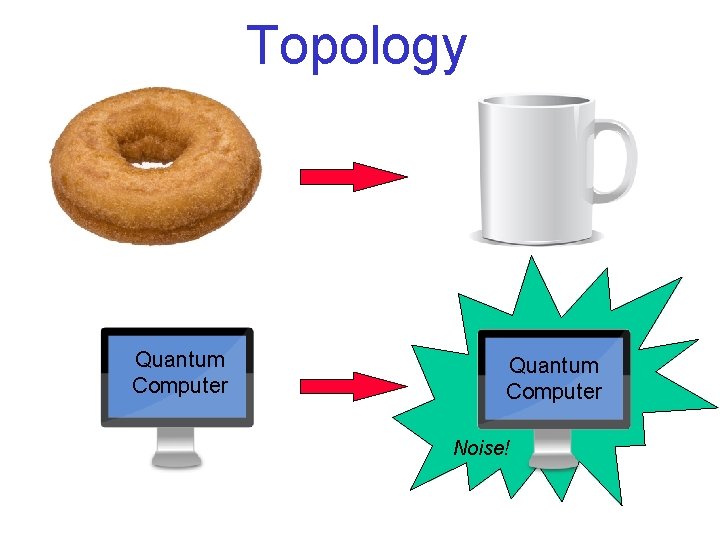
Topology Quantum Computer Noise!
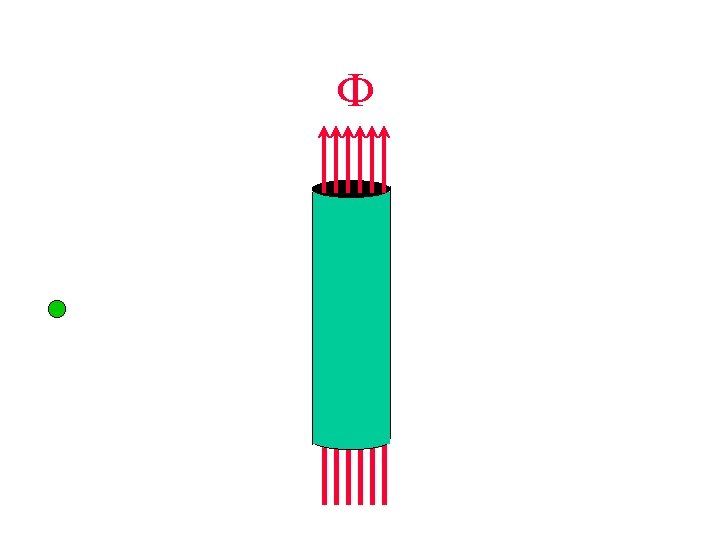
F
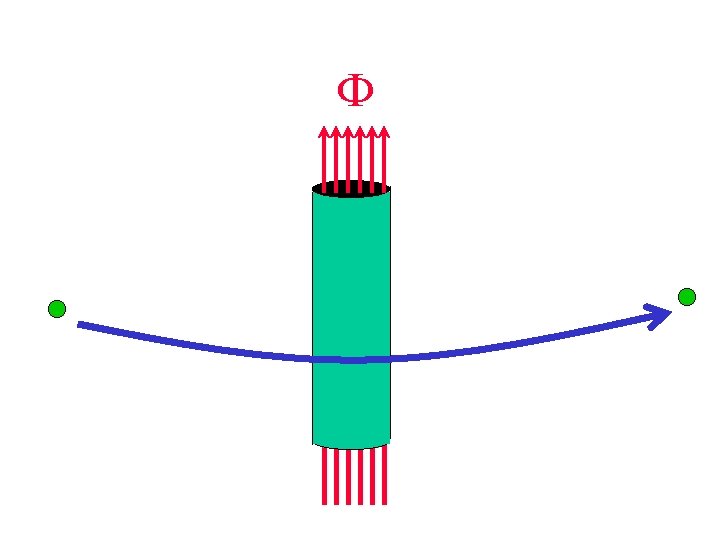
F
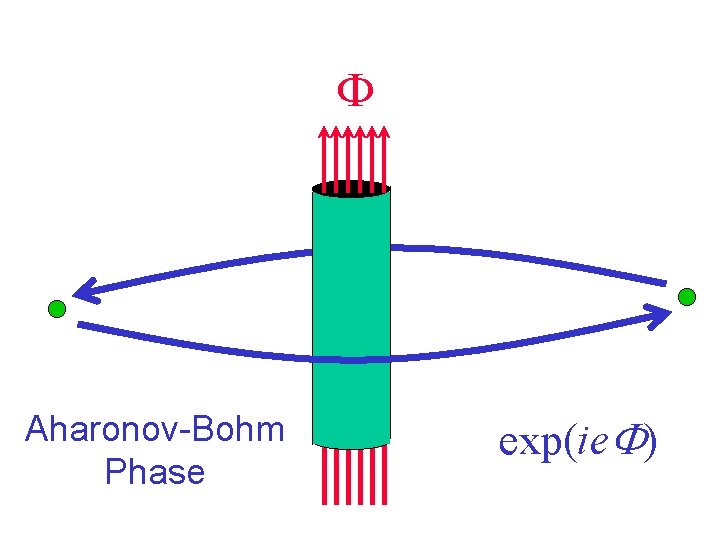
F Aharonov-Bohm Phase exp(ie. F)
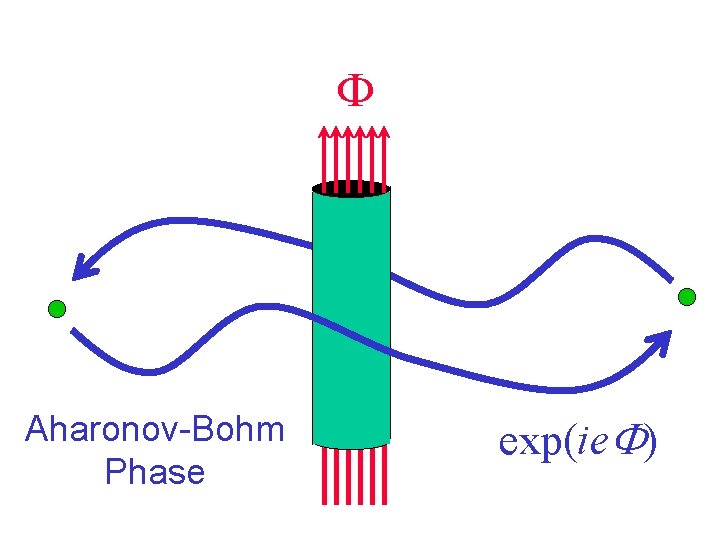
F Aharonov-Bohm Phase exp(ie. F)
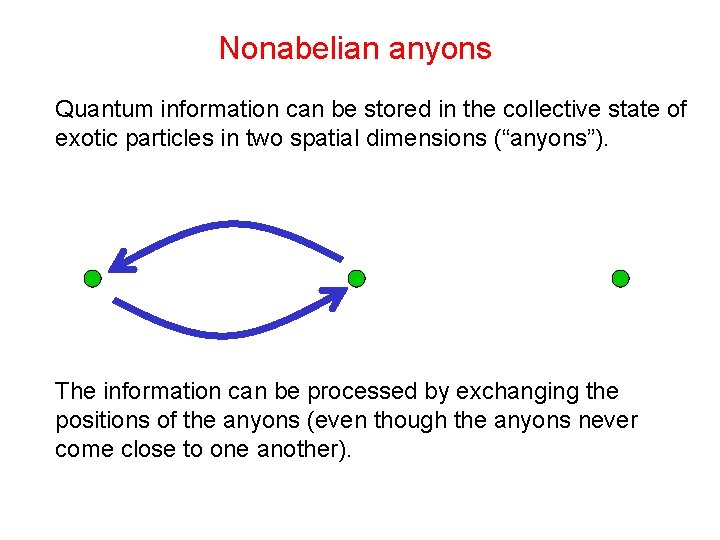
Nonabelian anyons Quantum information can be stored in the collective state of exotic particles in two spatial dimensions (“anyons”). The information can be processed by exchanging the positions of the anyons (even though the anyons never come close to one another).
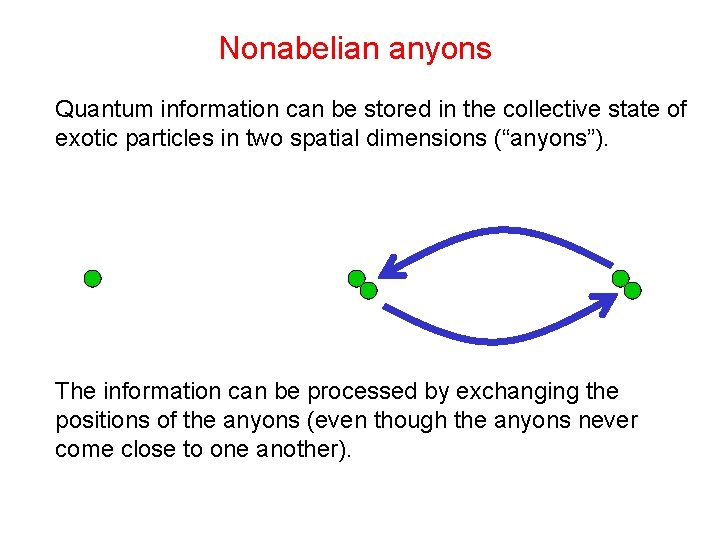
Nonabelian anyons Quantum information can be stored in the collective state of exotic particles in two spatial dimensions (“anyons”). The information can be processed by exchanging the positions of the anyons (even though the anyons never come close to one another).
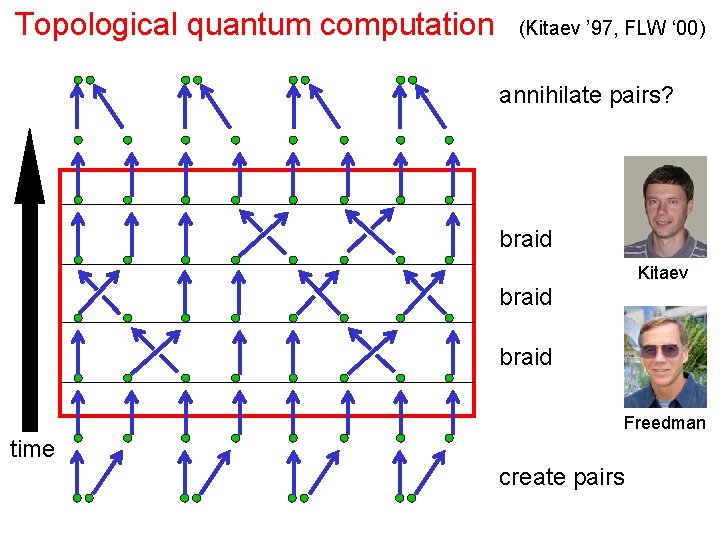
Topological quantum computation (Kitaev ’ 97, FLW ‘ 00) annihilate pairs? braid Kitaev braid Freedman time create pairs
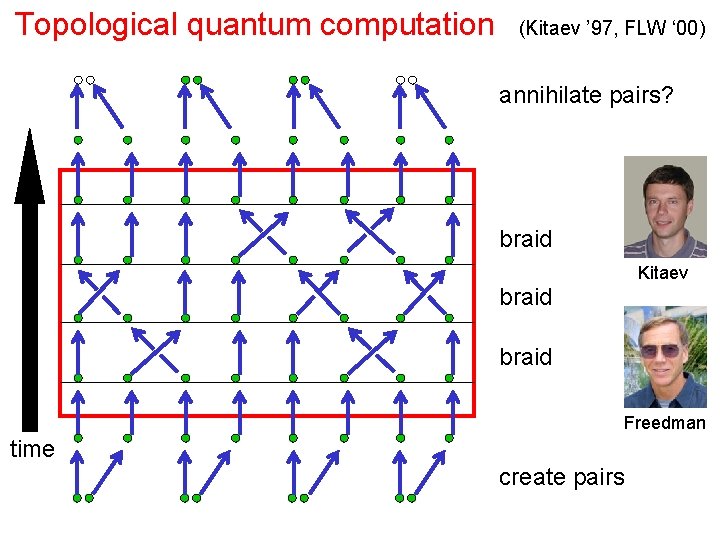
Topological quantum computation (Kitaev ’ 97, FLW ‘ 00) annihilate pairs? braid Kitaev braid Freedman time create pairs
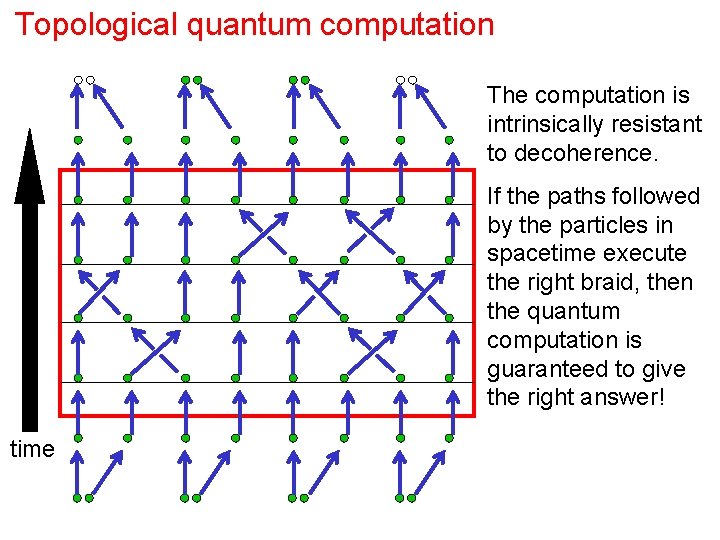
Topological quantum computation The computation is intrinsically resistant to decoherence. If the paths followed by the particles in spacetime execute the right braid, then the quantum computation is guaranteed to give the right answer! time
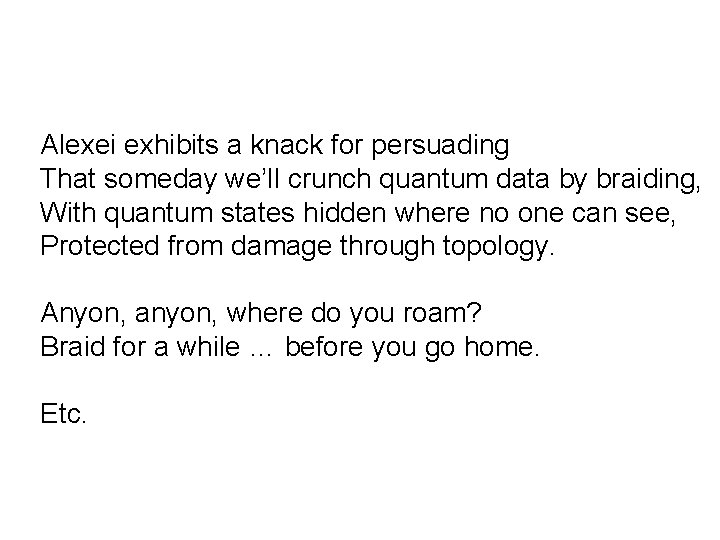
Alexei exhibits a knack for persuading That someday we’ll crunch quantum data by braiding, With quantum states hidden where no one can see, Protected from damage through topology. Anyon, anyon, where do you roam? Braid for a while … before you go home. Etc.
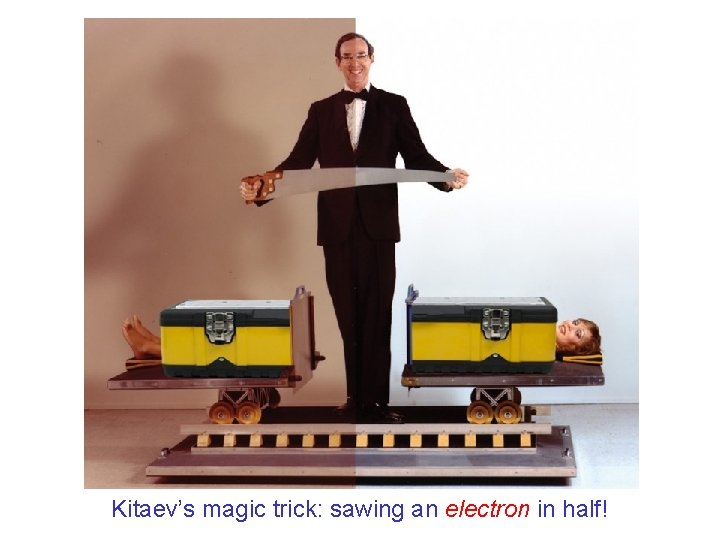
Kitaev’s magic trick: sawing an electron in half!
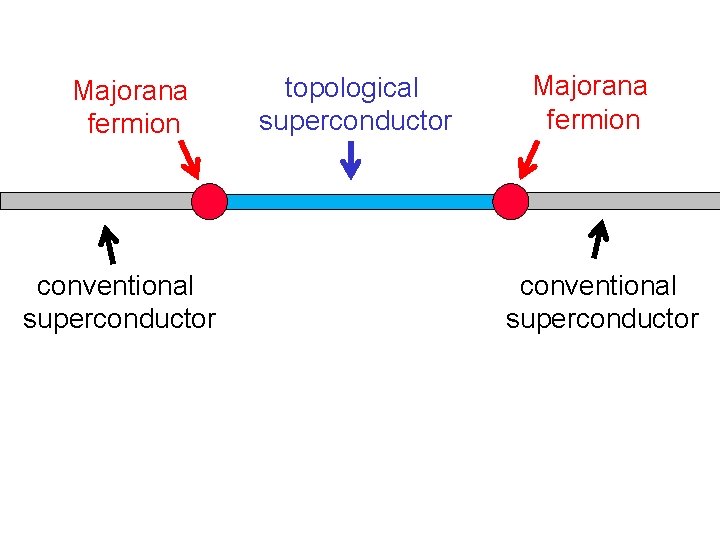
Majorana fermion conventional superconductor topological superconductor Majorana fermion conventional superconductor
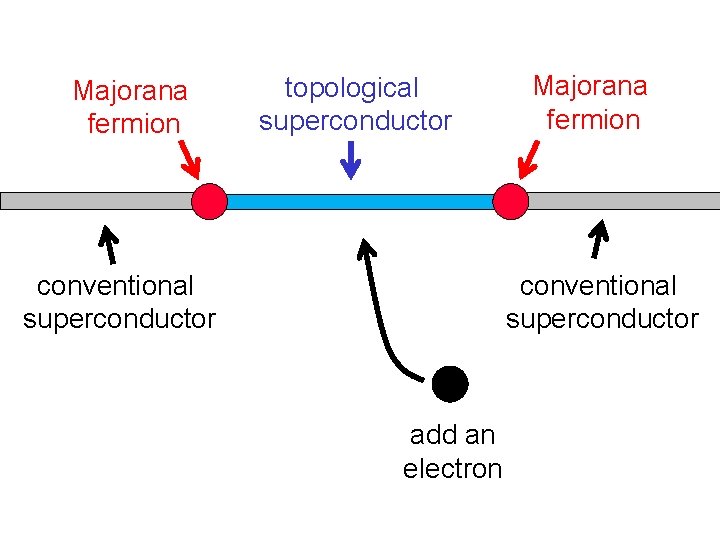
Majorana fermion topological superconductor Majorana fermion conventional superconductor add an electron
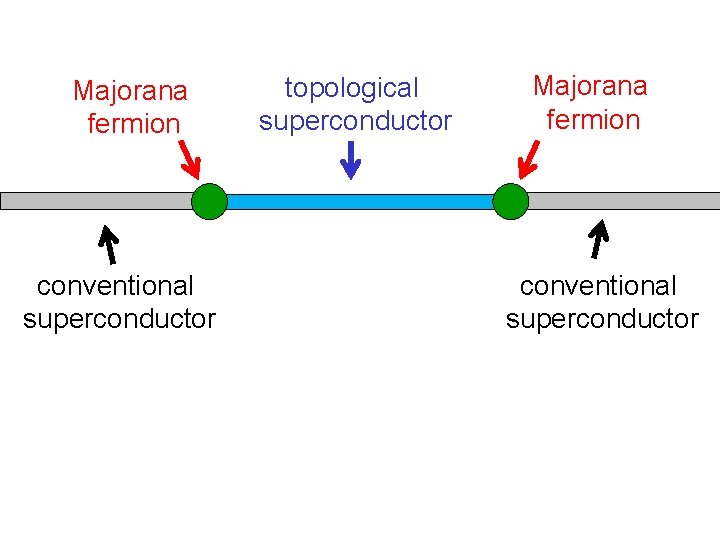
Majorana fermion conventional superconductor topological superconductor Majorana fermion conventional superconductor
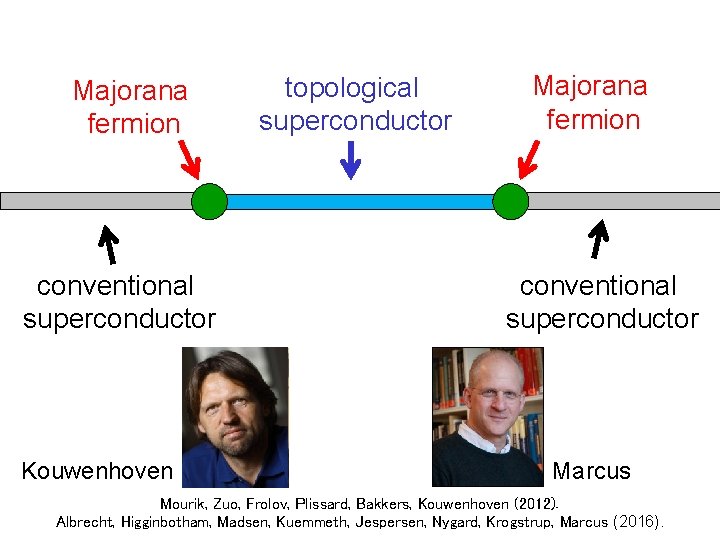
Majorana fermion conventional superconductor Kouwenhoven topological superconductor Majorana fermion conventional superconductor Marcus Mourik, Zuo, Frolov, Plissard, Bakkers, Kouwenhoven (2012). Albrecht, Higginbotham, Madsen, Kuemmeth, Jespersen, Nygard, Krogstrup, Marcus (2016).
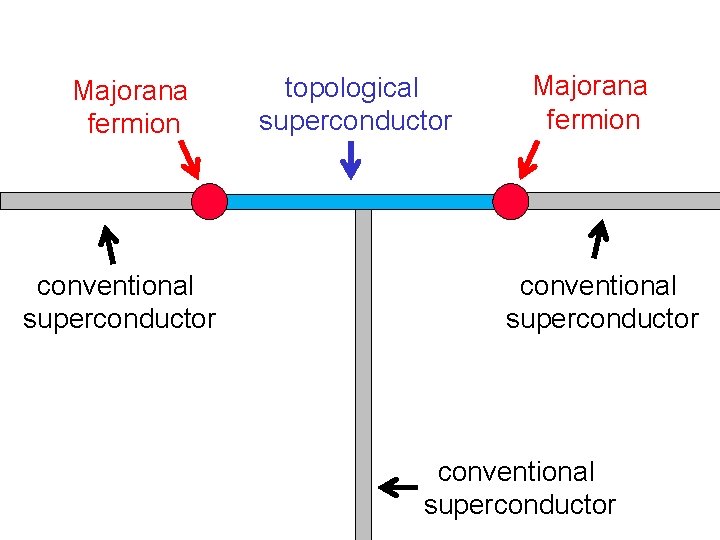
Majorana fermion conventional superconductor topological superconductor Majorana fermion conventional superconductor
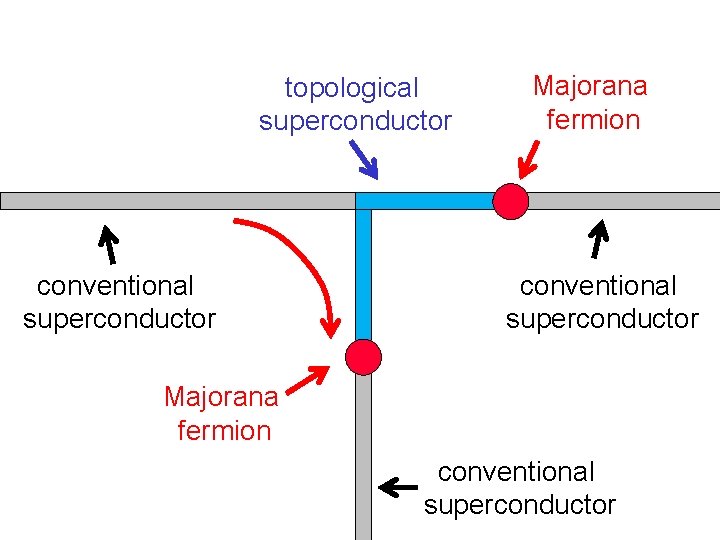
topological superconductor conventional superconductor Majorana fermion conventional superconductor
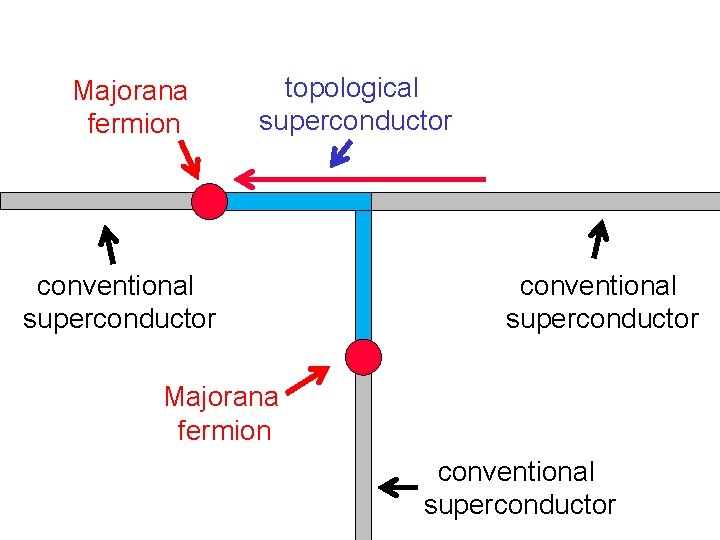
Majorana fermion topological superconductor conventional superconductor Majorana fermion conventional superconductor
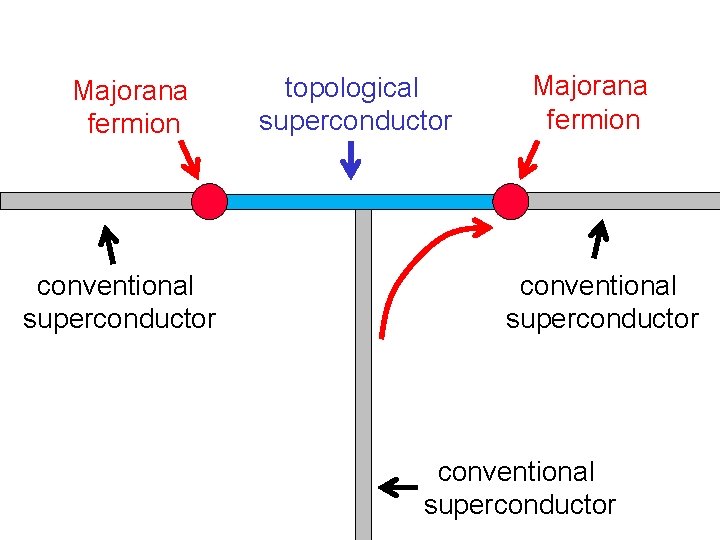
Majorana fermion conventional superconductor topological superconductor Majorana fermion conventional superconductor
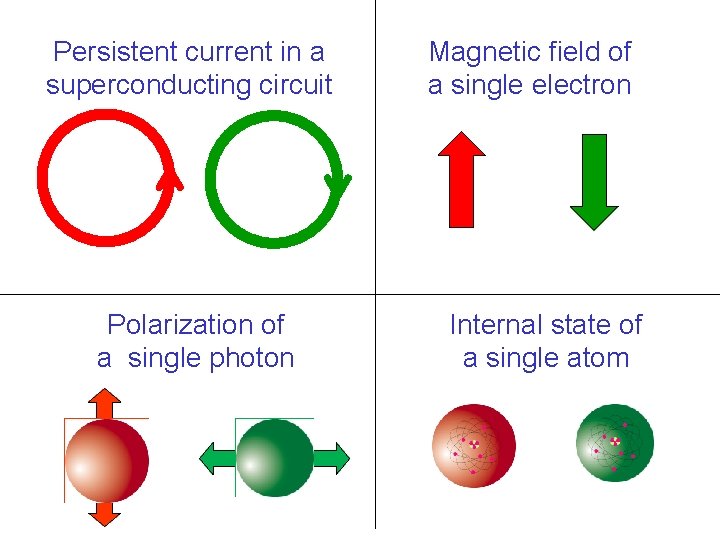
Persistent current in a superconducting circuit Polarization of a single photon Magnetic field of a single electron Internal state of a single atom
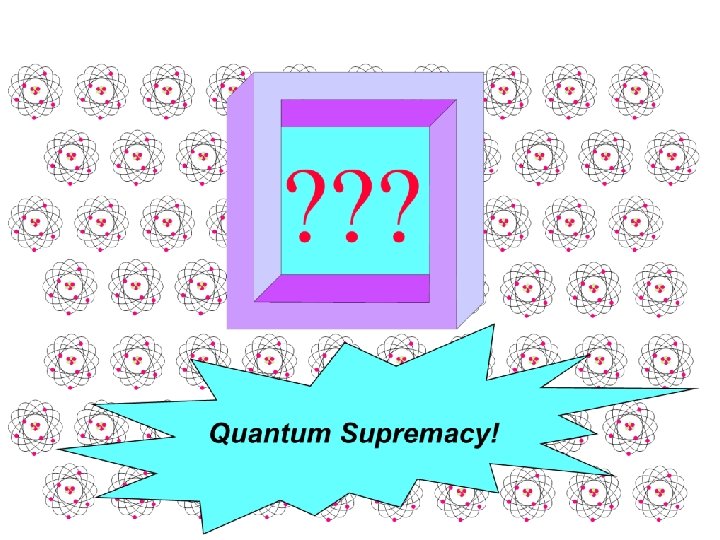
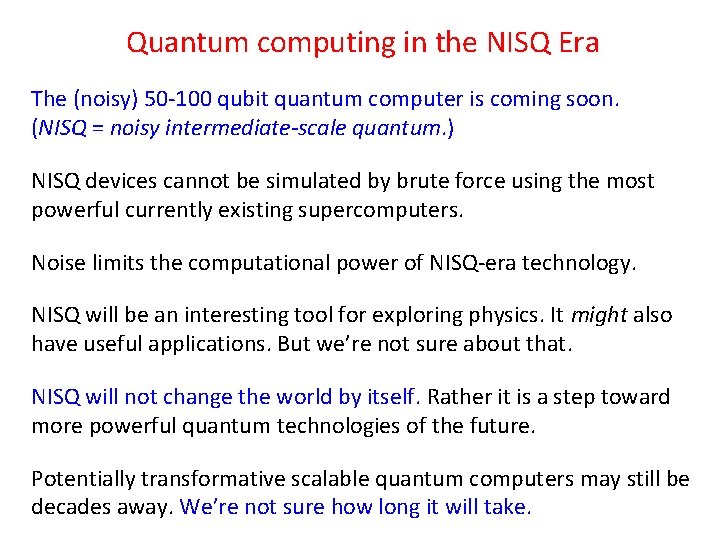
Quantum computing in the NISQ Era The (noisy) 50 -100 qubit quantum computer is coming soon. (NISQ = noisy intermediate-scale quantum. ) NISQ devices cannot be simulated by brute force using the most powerful currently existing supercomputers. Noise limits the computational power of NISQ-era technology. NISQ will be an interesting tool for exploring physics. It might also have useful applications. But we’re not sure about that. NISQ will not change the world by itself. Rather it is a step toward more powerful quantum technologies of the future. Potentially transformative scalable quantum computers may still be decades away. We’re not sure how long it will take.
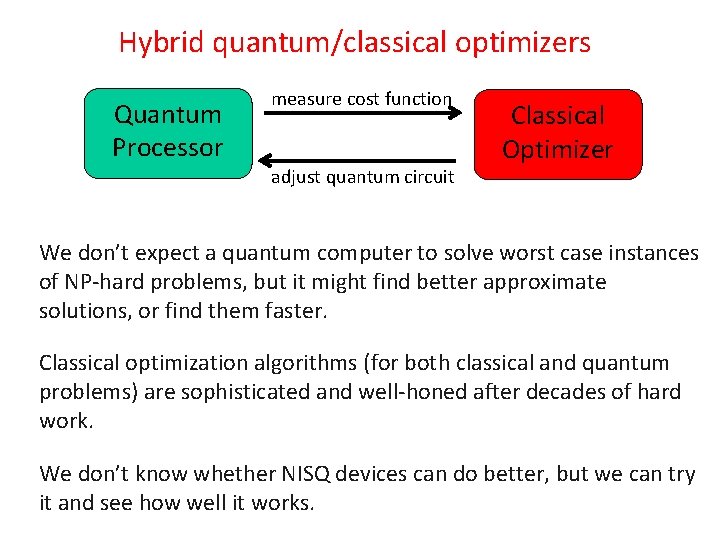
Hybrid quantum/classical optimizers Quantum Processor measure cost function adjust quantum circuit Classical Optimizer We don’t expect a quantum computer to solve worst case instances of NP-hard problems, but it might find better approximate solutions, or find them faster. Classical optimization algorithms (for both classical and quantum problems) are sophisticated and well-honed after decades of hard work. We don’t know whether NISQ devices can do better, but we can try it and see how well it works.
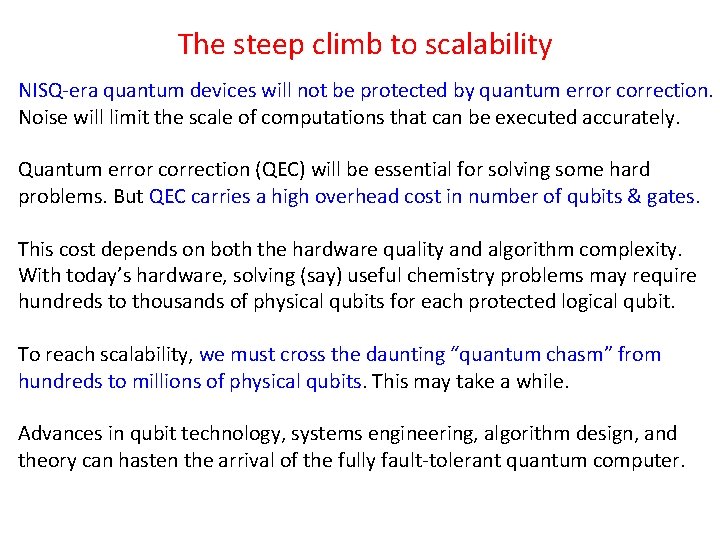
The steep climb to scalability NISQ-era quantum devices will not be protected by quantum error correction. Noise will limit the scale of computations that can be executed accurately. Quantum error correction (QEC) will be essential for solving some hard problems. But QEC carries a high overhead cost in number of qubits & gates. This cost depends on both the hardware quality and algorithm complexity. With today’s hardware, solving (say) useful chemistry problems may require hundreds to thousands of physical qubits for each protected logical qubit. To reach scalability, we must cross the daunting “quantum chasm” from hundreds to millions of physical qubits. This may take a while. Advances in qubit technology, systems engineering, algorithm design, and theory can hasten the arrival of the fully fault-tolerant quantum computer.
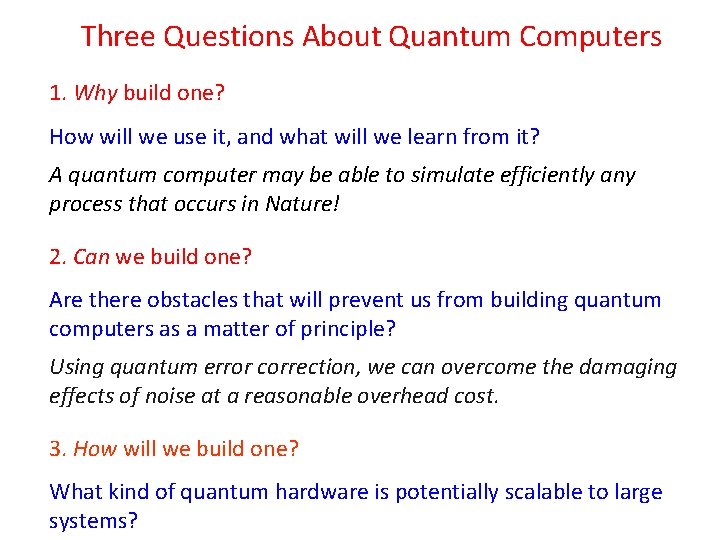
Three Questions About Quantum Computers 1. Why build one? How will we use it, and what will we learn from it? A quantum computer may be able to simulate efficiently any process that occurs in Nature! 2. Can we build one? Are there obstacles that will prevent us from building quantum computers as a matter of principle? Using quantum error correction, we can overcome the damaging effects of noise at a reasonable overhead cost. 3. How will we build one? What kind of quantum hardware is potentially scalable to large systems?
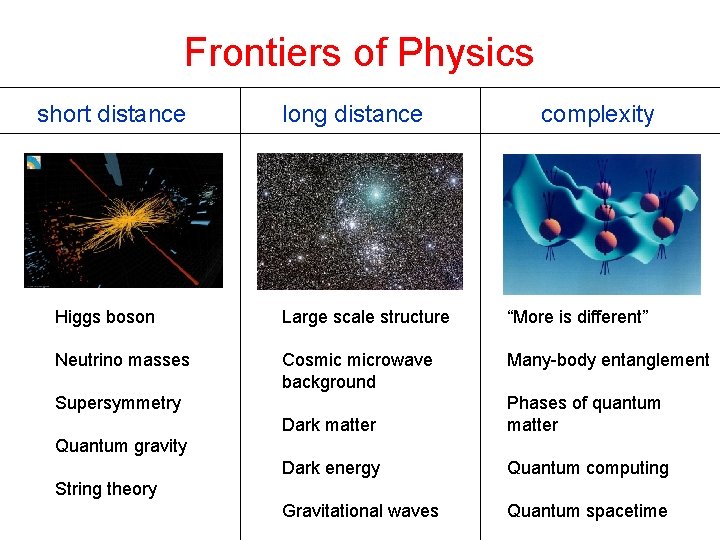
Frontiers of Physics short distance long distance complexity Higgs boson Large scale structure “More is different” Neutrino masses Cosmic microwave background Many-body entanglement Supersymmetry Dark matter Phases of quantum matter Dark energy Quantum computing Gravitational waves Quantum spacetime Quantum gravity String theory
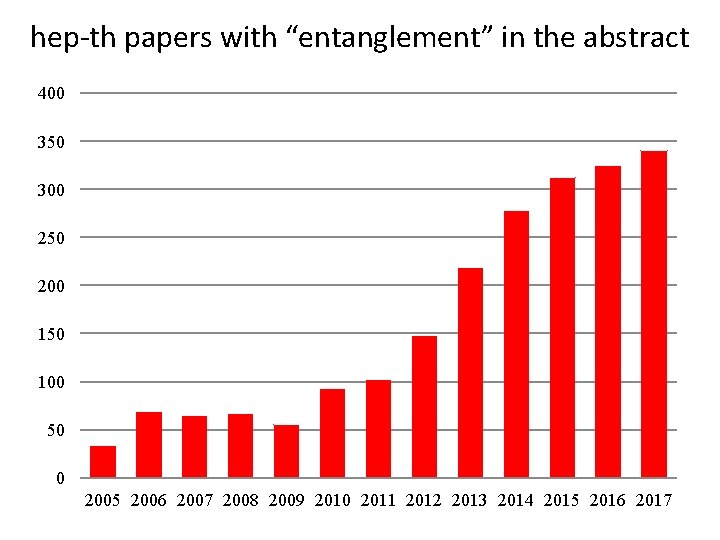
hep-th papers with “entanglement” in the abstract 400 350 300 250 200 150 100 50 0 2005 2006 2007 2008 2009 2010 2011 2012 2013 2014 2015 2016 2017
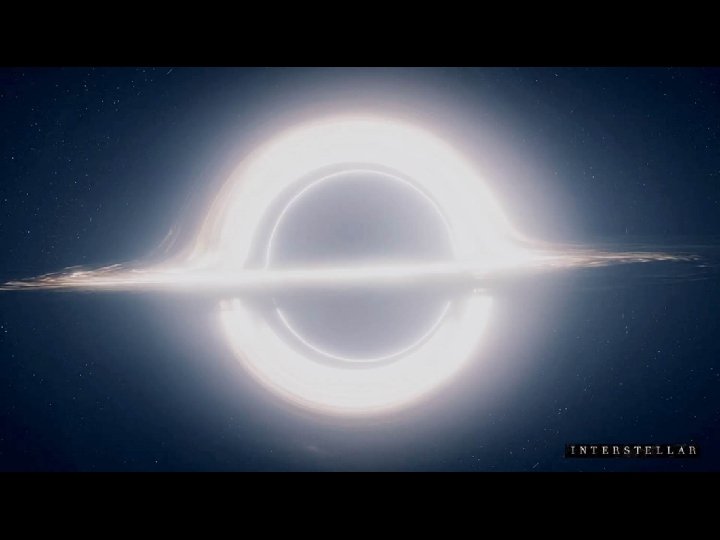
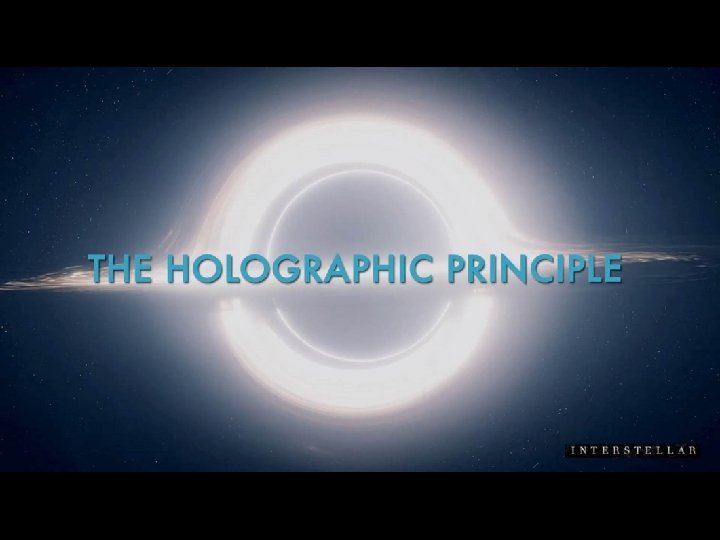
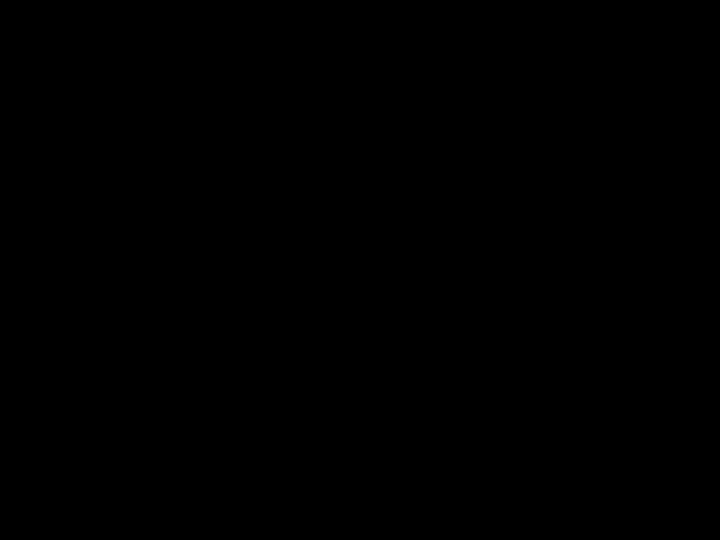
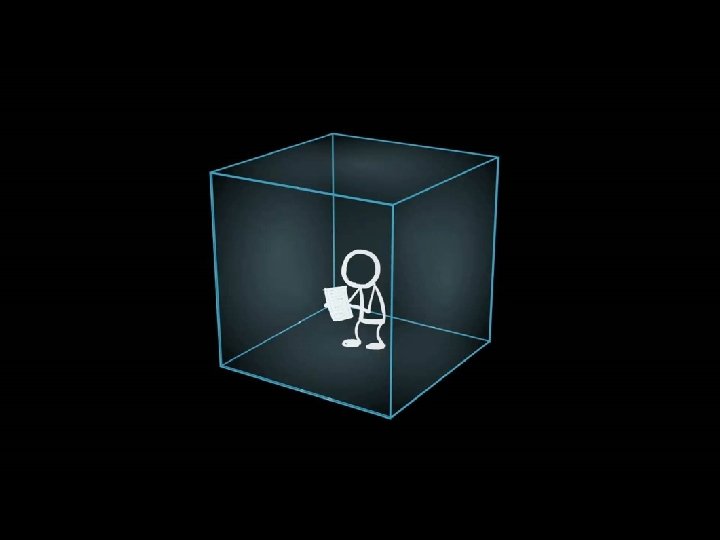
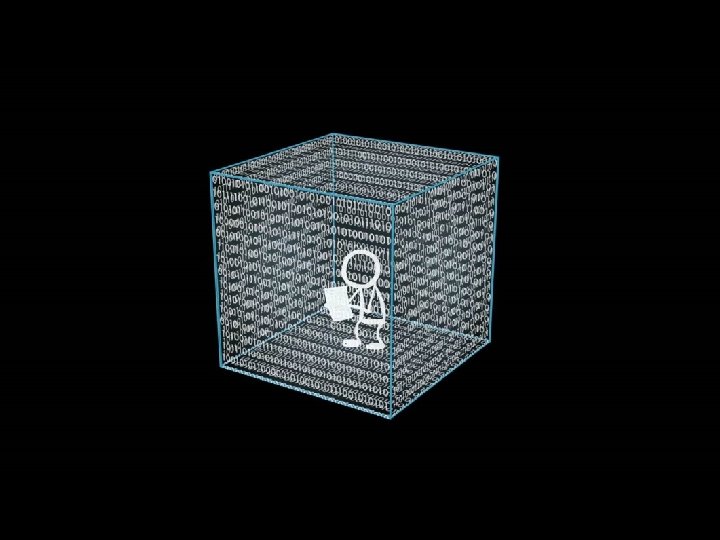
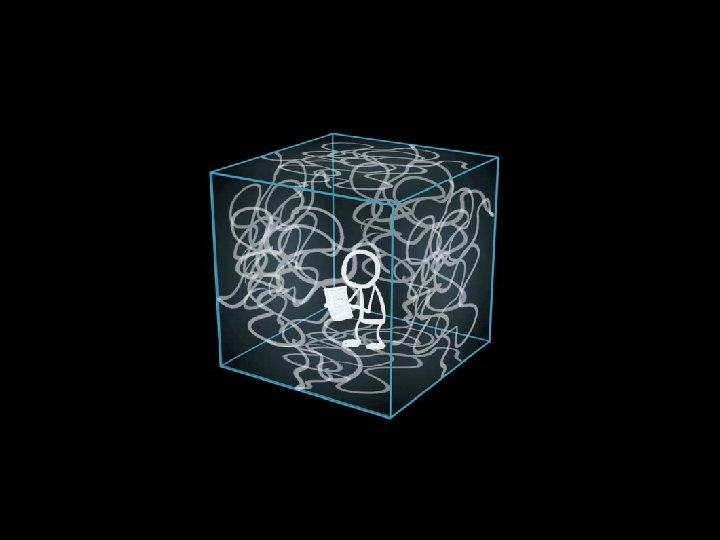
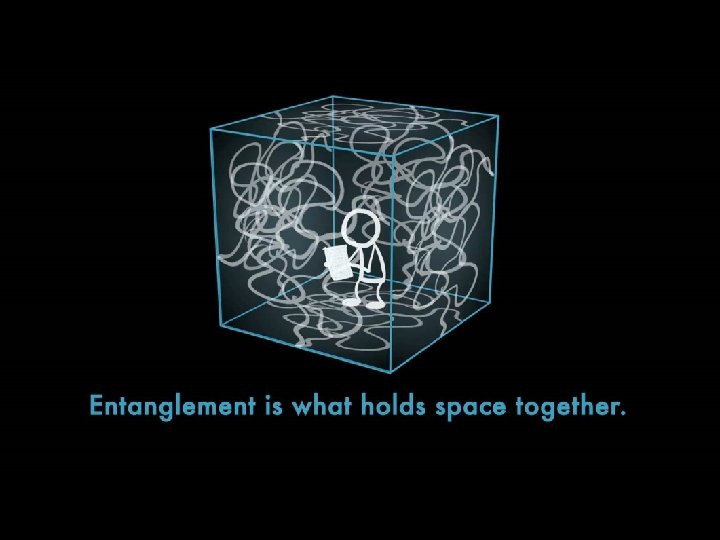
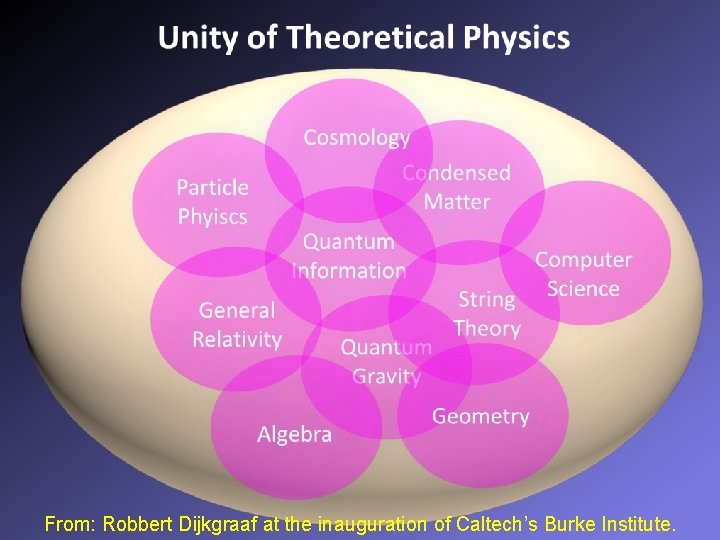
From: Robbert Dijkgraaf at the inauguration of Caltech’s Burke Institute.
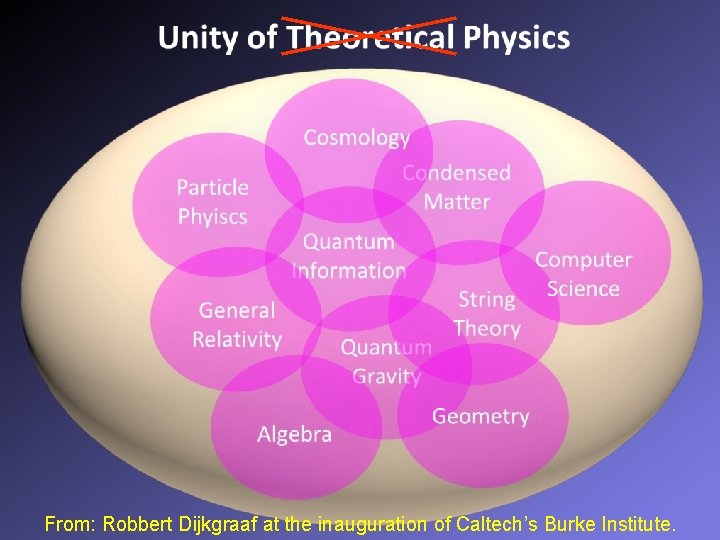
From: Robbert Dijkgraaf at the inauguration of Caltech’s Burke Institute.
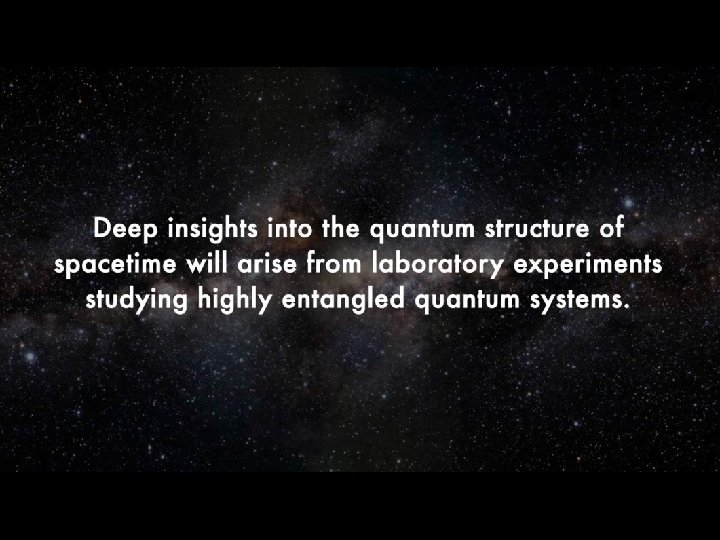
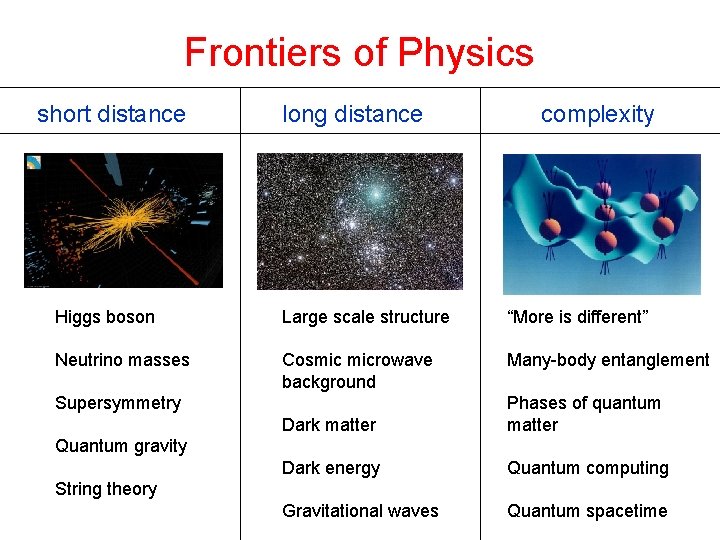
Frontiers of Physics short distance long distance complexity Higgs boson Large scale structure “More is different” Neutrino masses Cosmic microwave background Many-body entanglement Supersymmetry Dark matter Phases of quantum matter Dark energy Quantum computing Gravitational waves Quantum spacetime Quantum gravity String theory
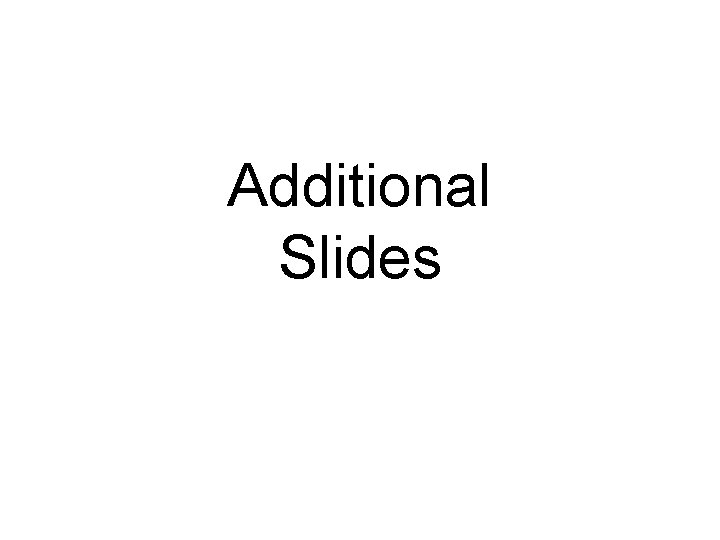
Additional Slides
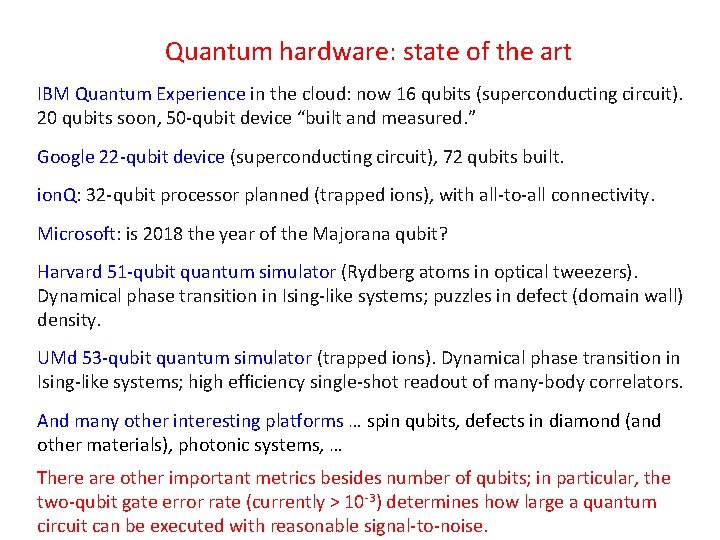
Quantum hardware: state of the art IBM Quantum Experience in the cloud: now 16 qubits (superconducting circuit). 20 qubits soon, 50 -qubit device “built and measured. ” Google 22 -qubit device (superconducting circuit), 72 qubits built. ion. Q: 32 -qubit processor planned (trapped ions), with all-to-all connectivity. Microsoft: is 2018 the year of the Majorana qubit? Harvard 51 -qubit quantum simulator (Rydberg atoms in optical tweezers). Dynamical phase transition in Ising-like systems; puzzles in defect (domain wall) density. UMd 53 -qubit quantum simulator (trapped ions). Dynamical phase transition in Ising-like systems; high efficiency single-shot readout of many-body correlators. And many other interesting platforms … spin qubits, defects in diamond (and other materials), photonic systems, … There are other important metrics besides number of qubits; in particular, the two-qubit gate error rate (currently > 10 -3) determines how large a quantum circuit can be executed with reasonable signal-to-noise.
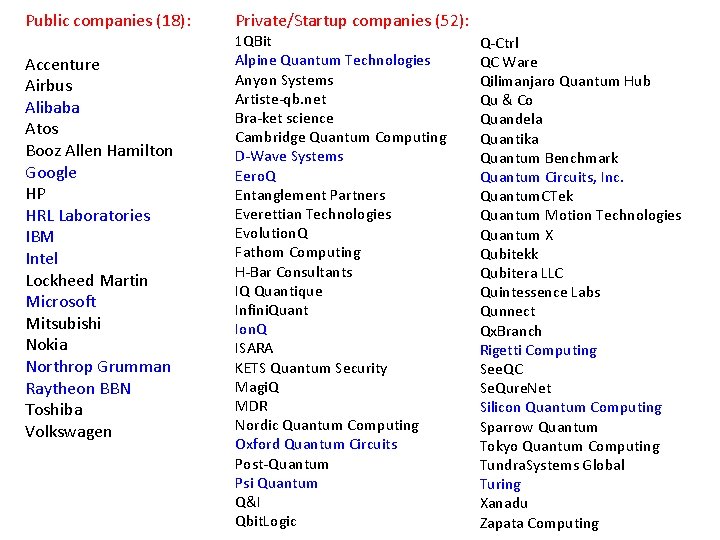
Public companies (18): Accenture Airbus Alibaba Atos Booz Allen Hamilton Google HP HRL Laboratories IBM Intel Lockheed Martin Microsoft Mitsubishi Nokia Northrop Grumman Raytheon BBN Toshiba Volkswagen Private/Startup companies (52): 1 QBit Alpine Quantum Technologies Anyon Systems Artiste-qb. net Bra-ket science Cambridge Quantum Computing D-Wave Systems Eero. Q Entanglement Partners Everettian Technologies Evolution. Q Fathom Computing H-Bar Consultants IQ Quantique Infini. Quant Ion. Q ISARA KETS Quantum Security Magi. Q MDR Nordic Quantum Computing Oxford Quantum Circuits Post-Quantum Psi Quantum Q&I Qbit. Logic Q-Ctrl QC Ware Qilimanjaro Quantum Hub Qu & Co Quandela Quantika Quantum Benchmark Quantum Circuits, Inc. Quantum. CTek Quantum Motion Technologies Quantum X Qubitekk Qubitera LLC Quintessence Labs Qunnect Qx. Branch Rigetti Computing See. QC Se. Qure. Net Silicon Quantum Computing Sparrow Quantum Tokyo Quantum Computing Tundra. Systems Global Turing Xanadu Zapata Computing
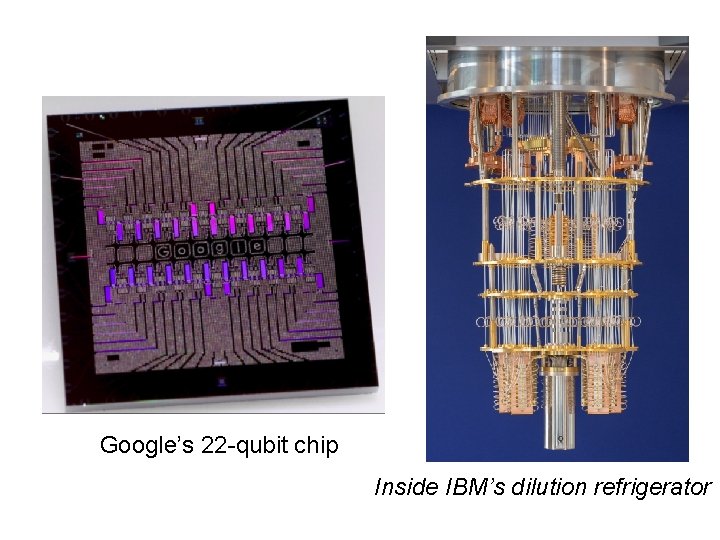
Google’s 22 -qubit chip Inside IBM’s dilution refrigerator
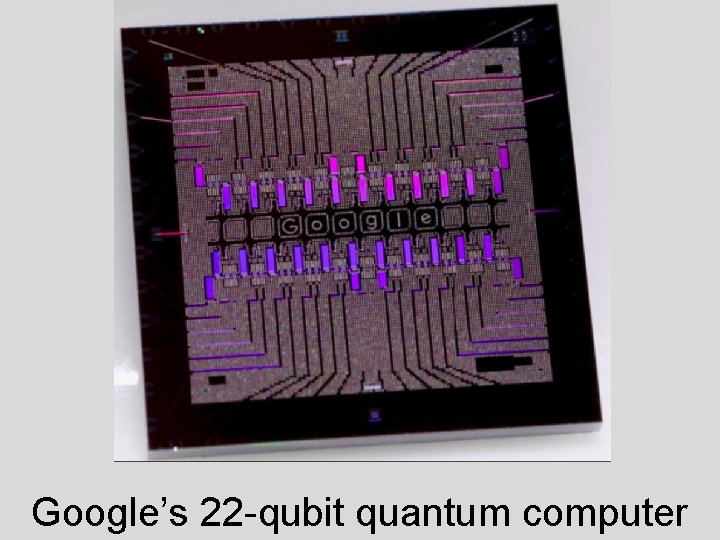
Google’s 22 -qubit quantum computer
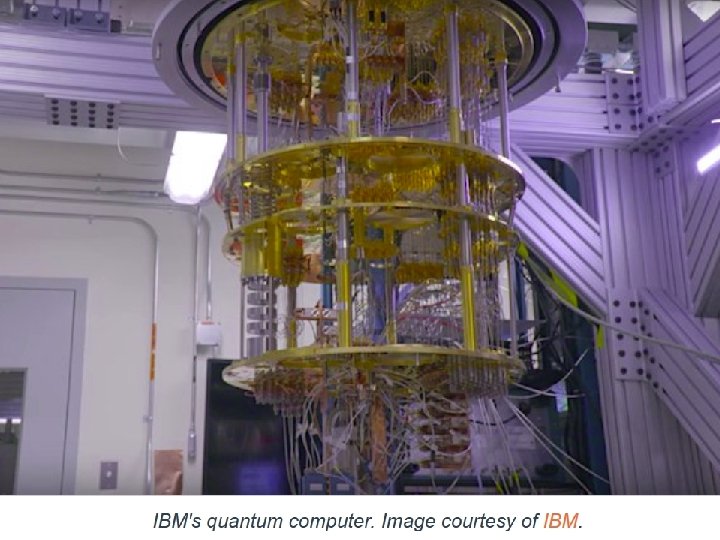
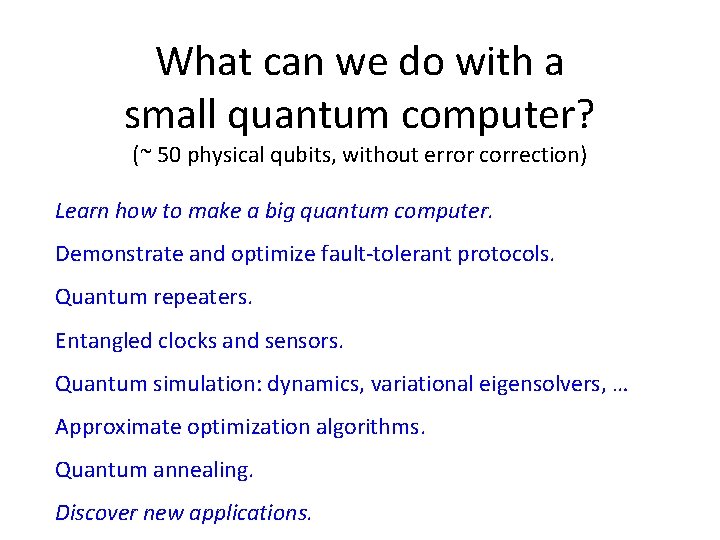
What can we do with a small quantum computer? (~ 50 physical qubits, without error correction) Learn how to make a big quantum computer. Demonstrate and optimize fault-tolerant protocols. Quantum repeaters. Entangled clocks and sensors. Quantum simulation: dynamics, variational eigensolvers, … Approximate optimization algorithms. Quantum annealing. Discover new applications.