Introduction to Material Engineering THE MARS ROVERS SPIRIT
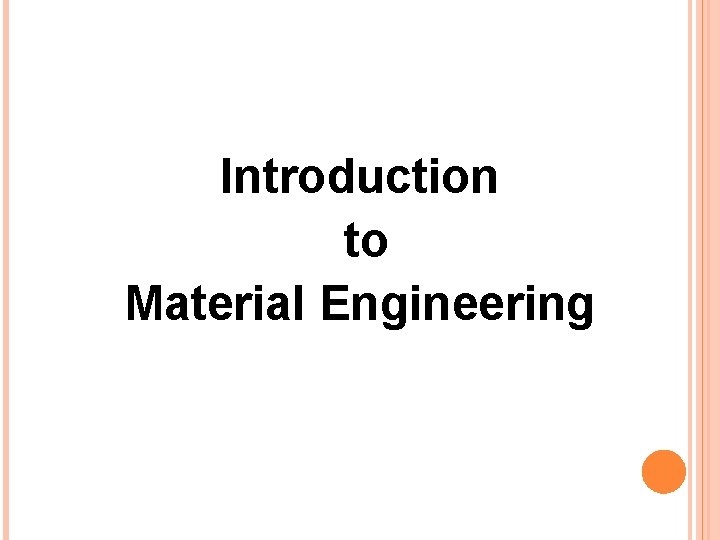
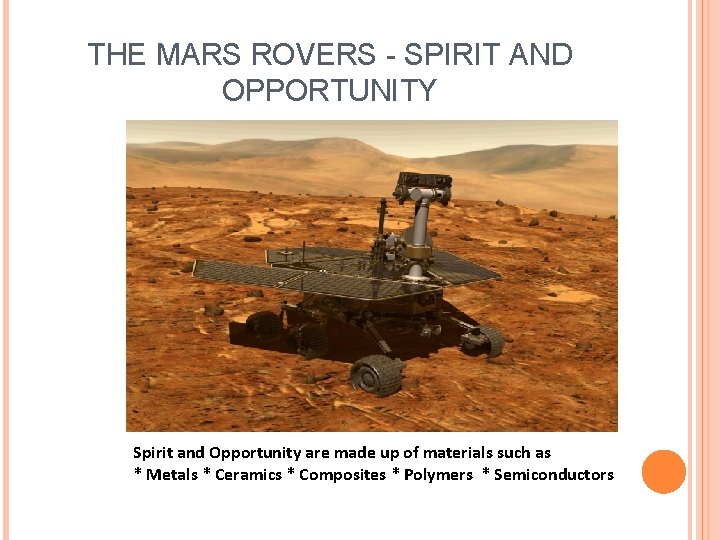
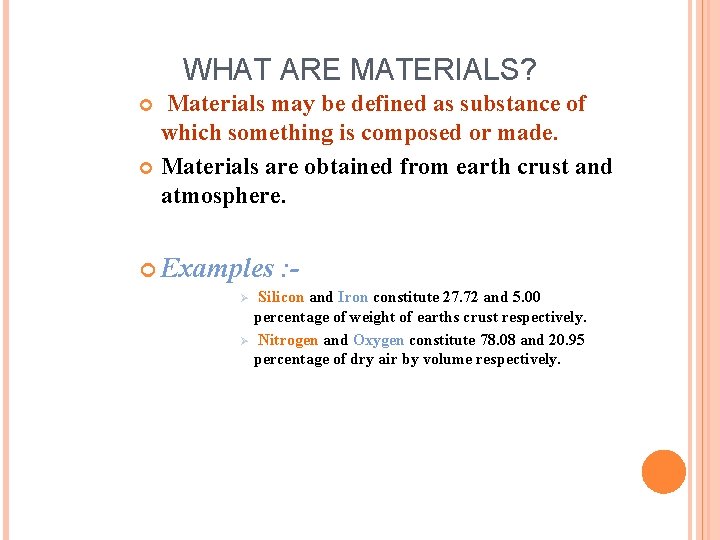
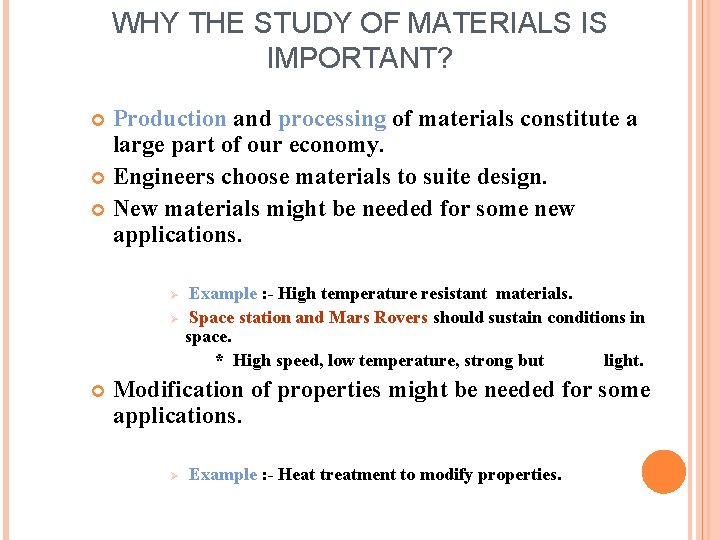
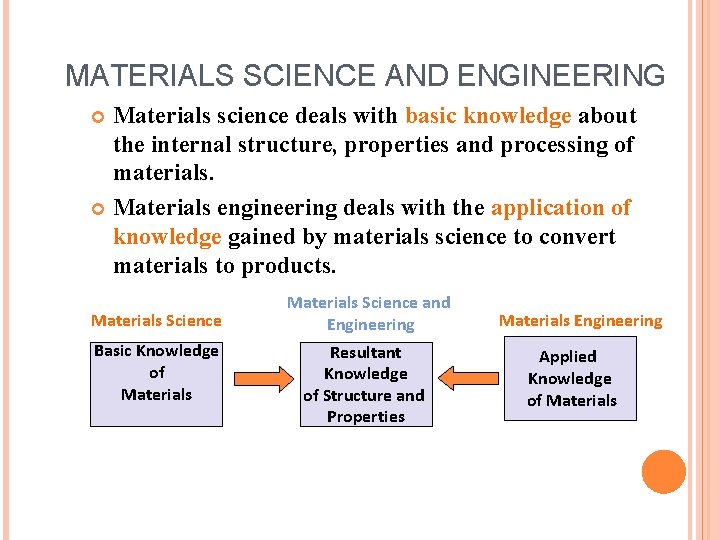
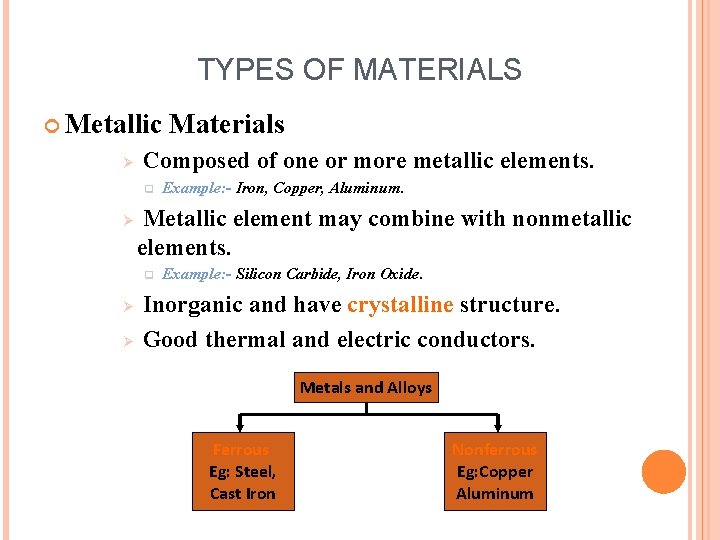
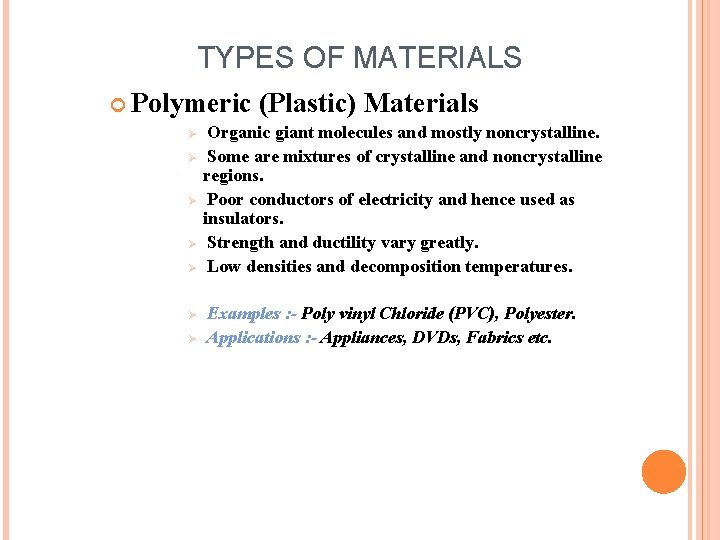
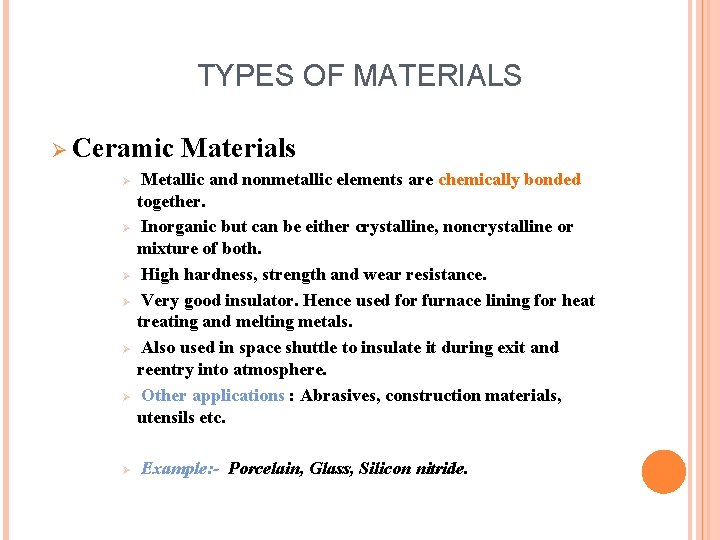
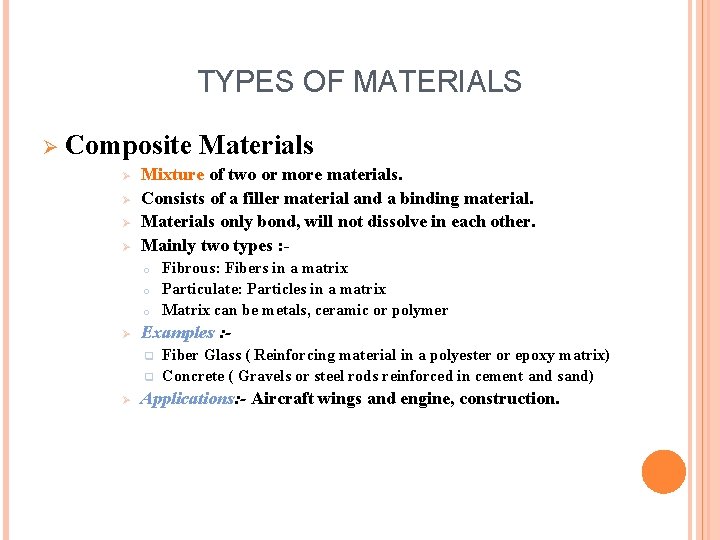
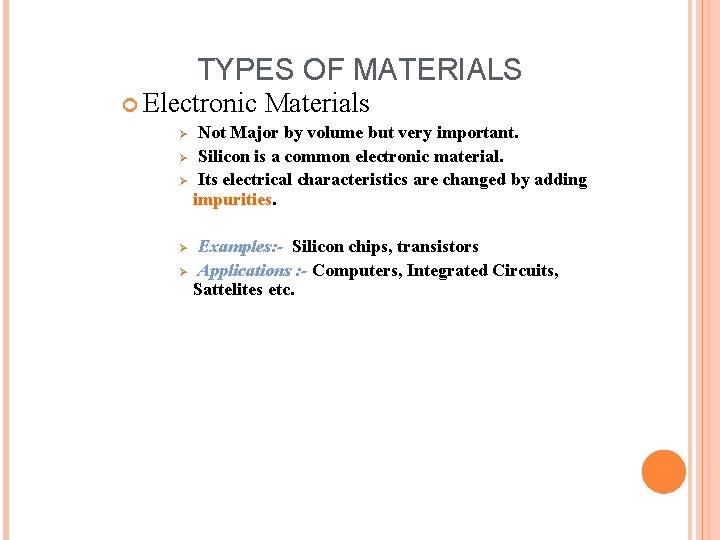
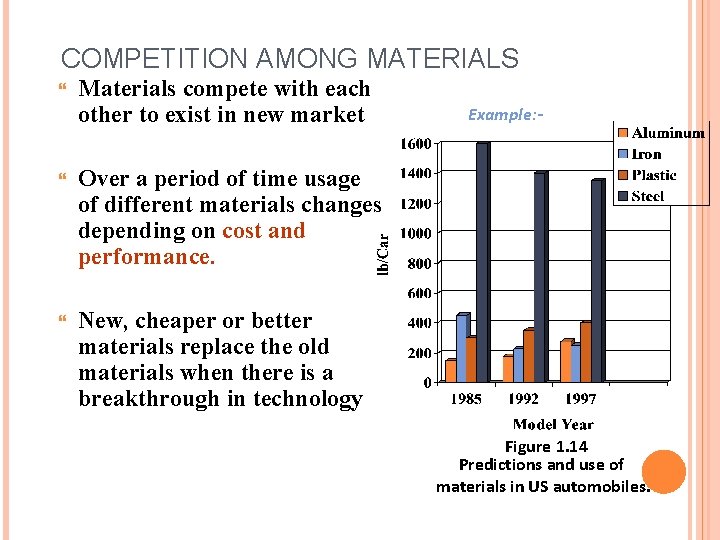
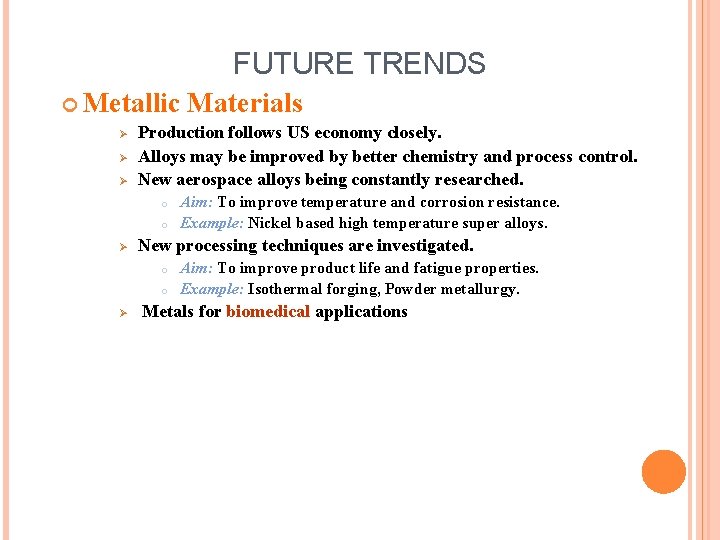
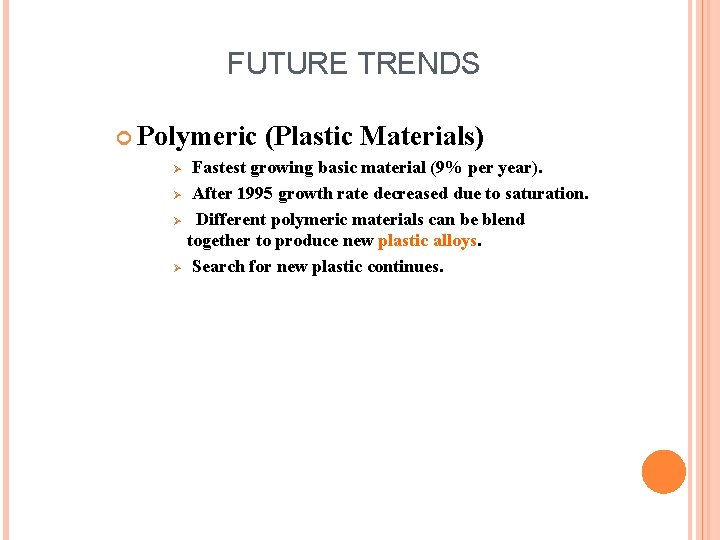
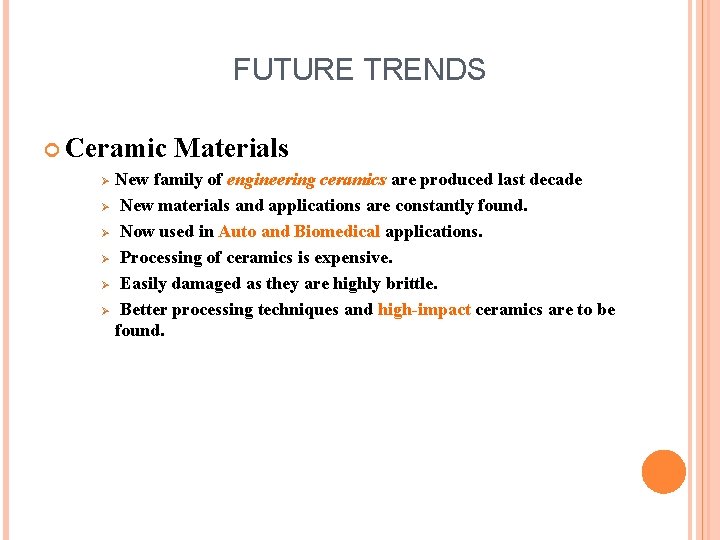
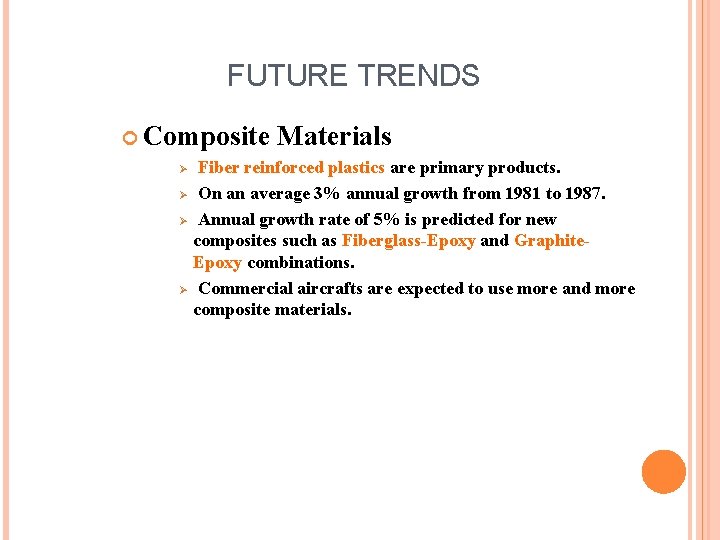
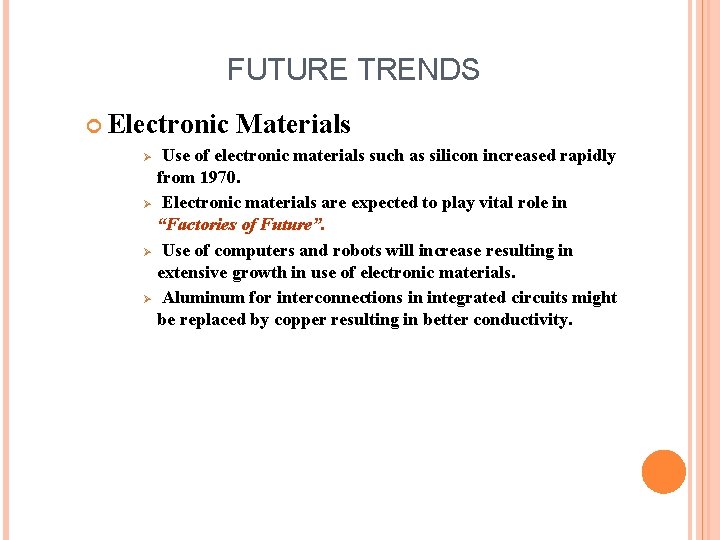
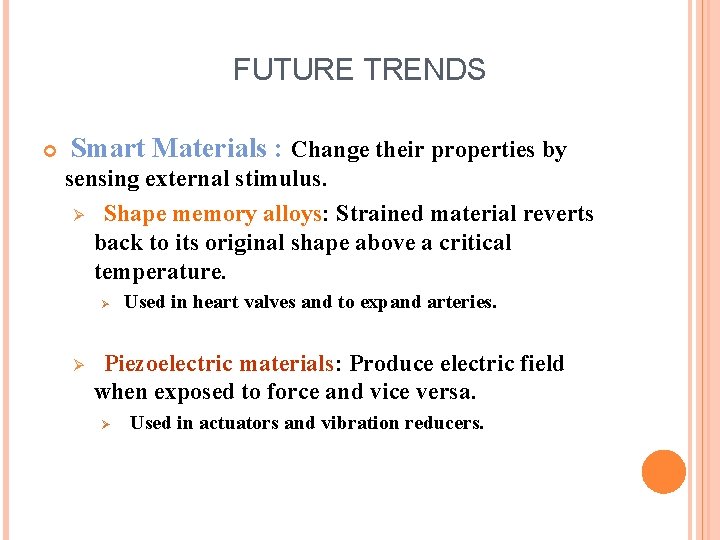
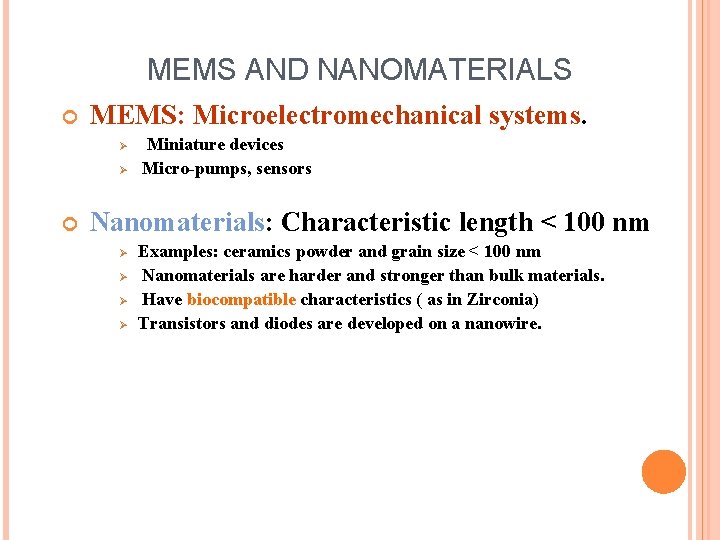
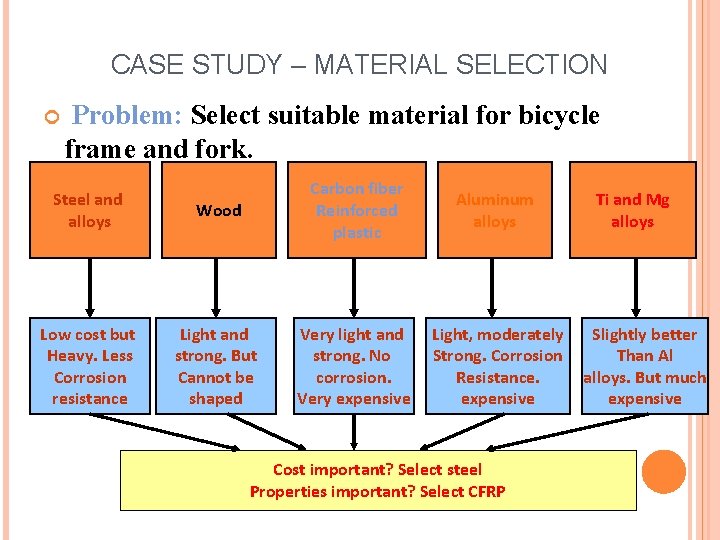
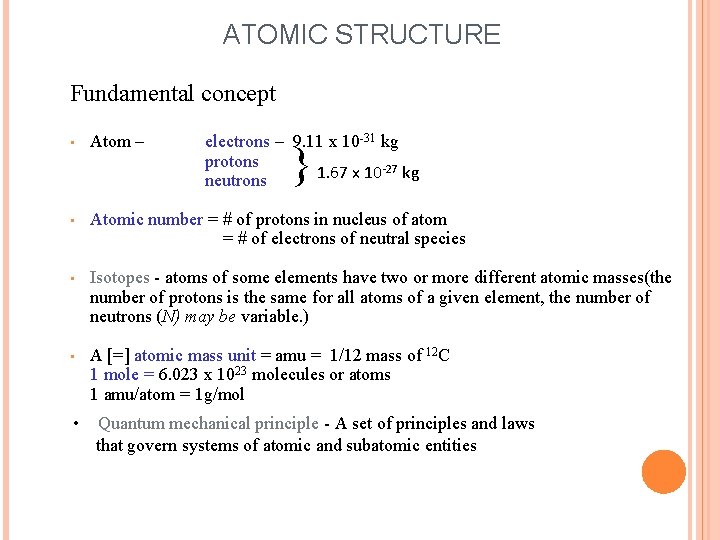
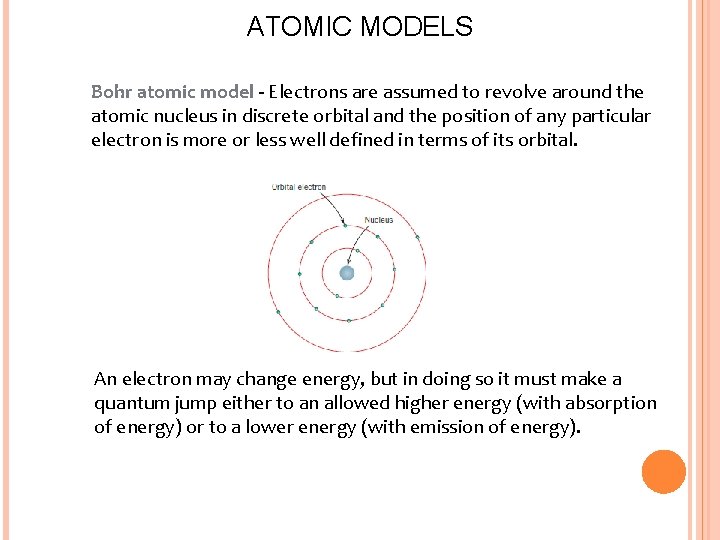
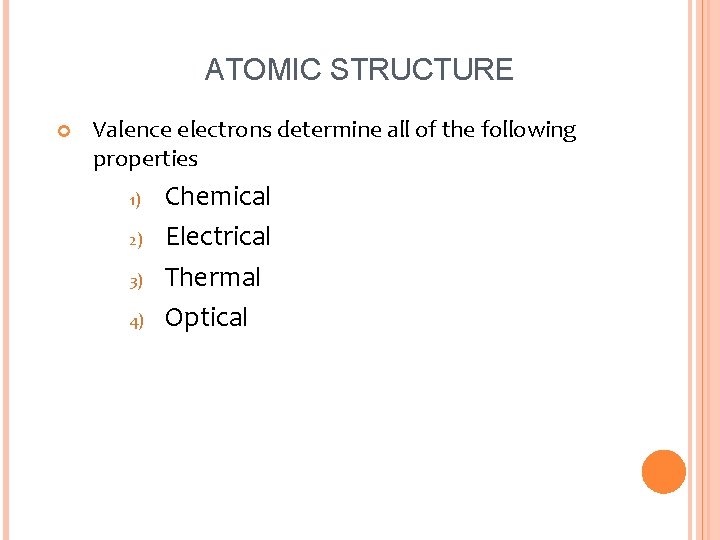
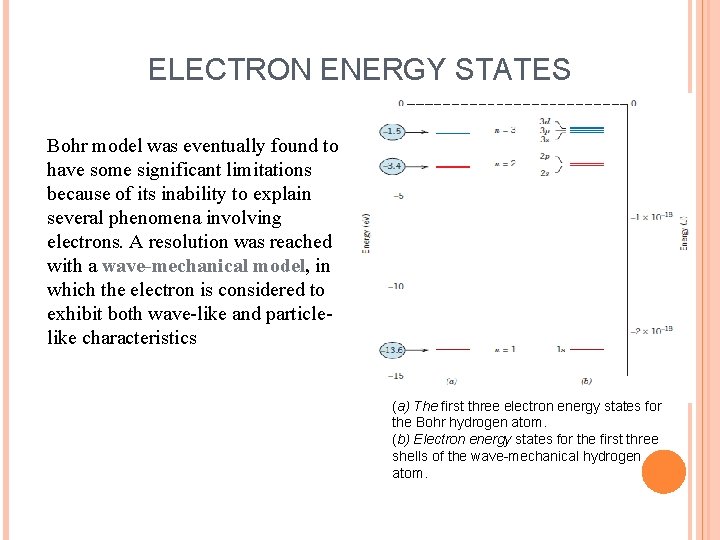
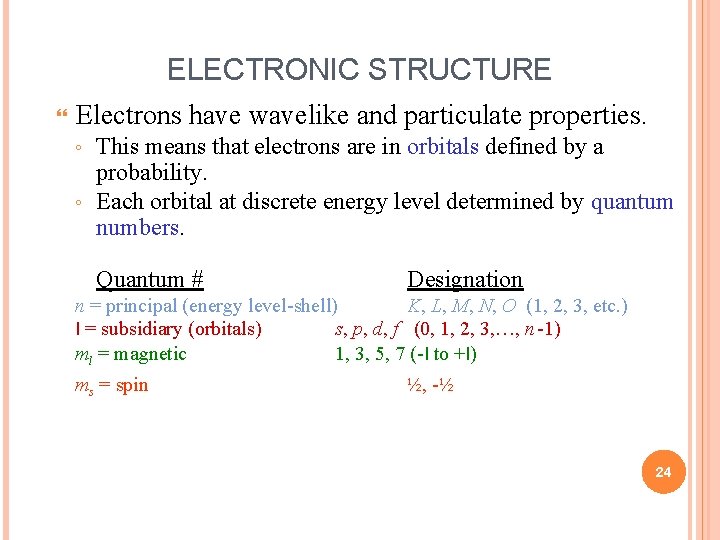
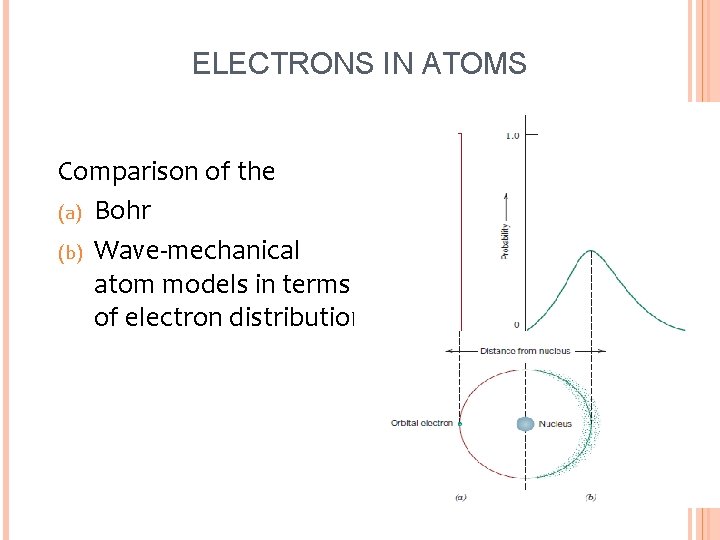
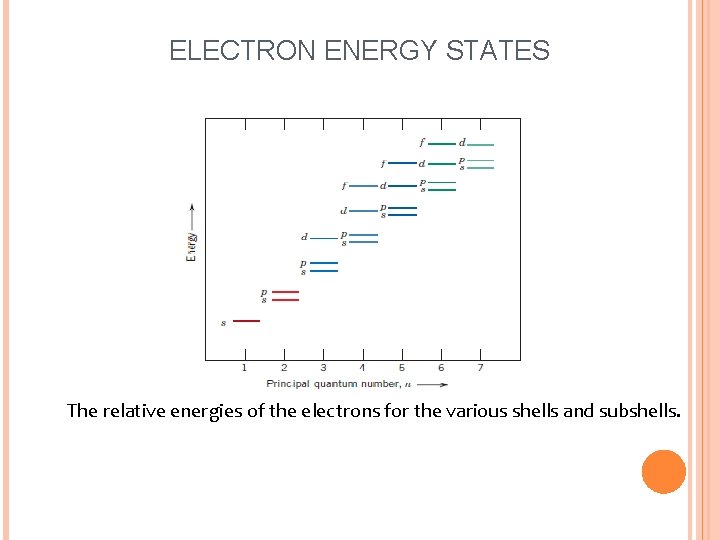
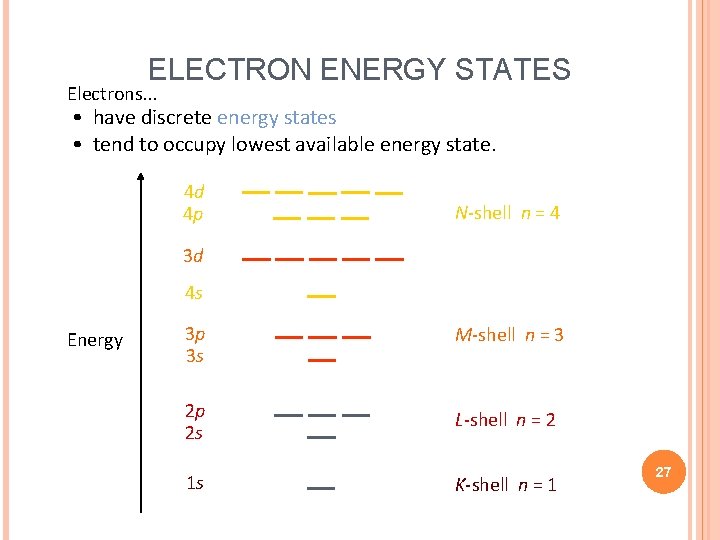
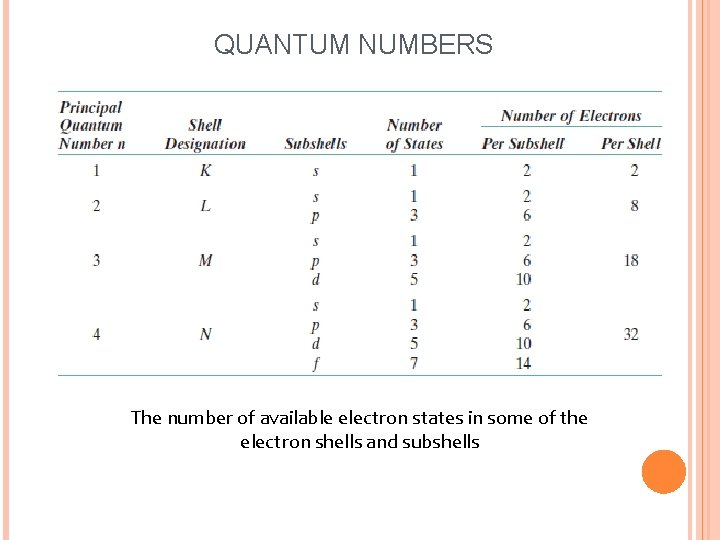
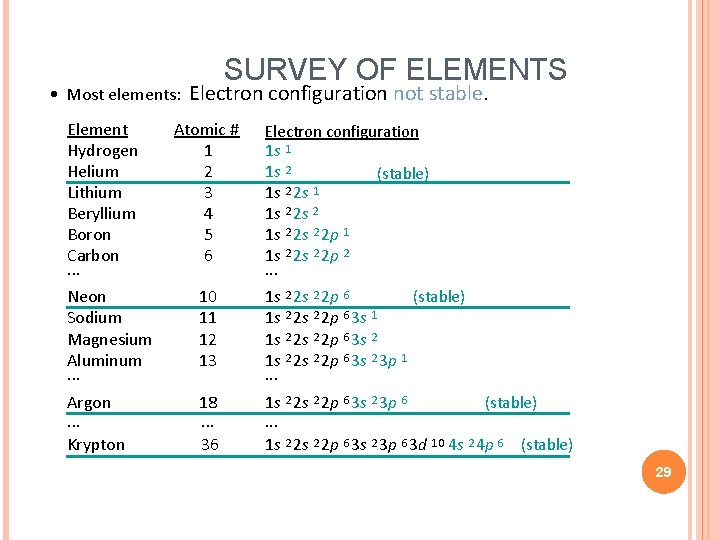
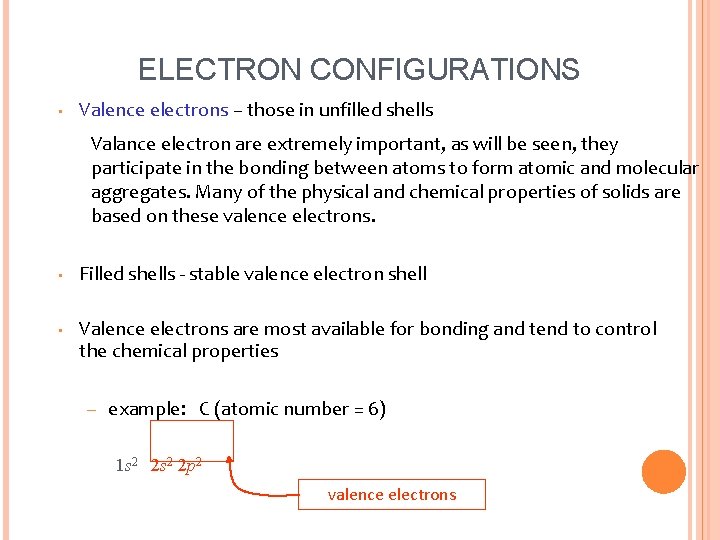
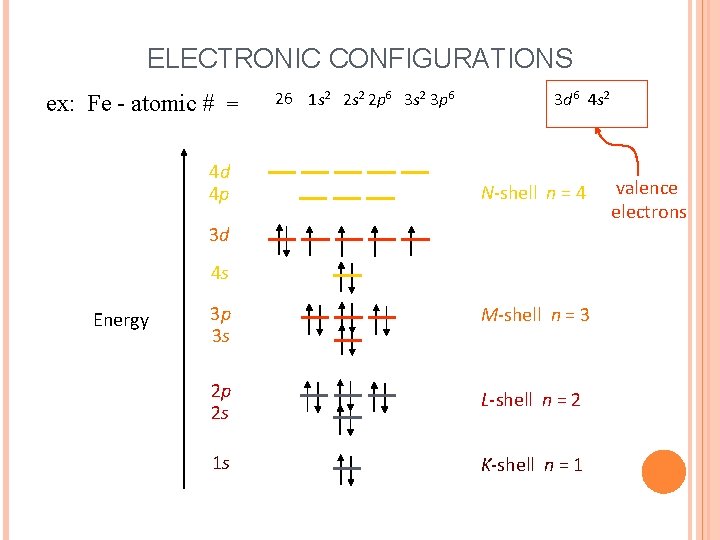
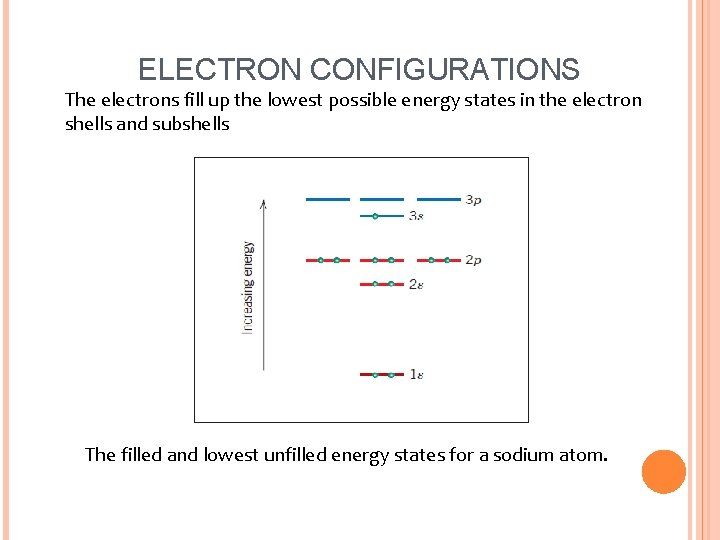
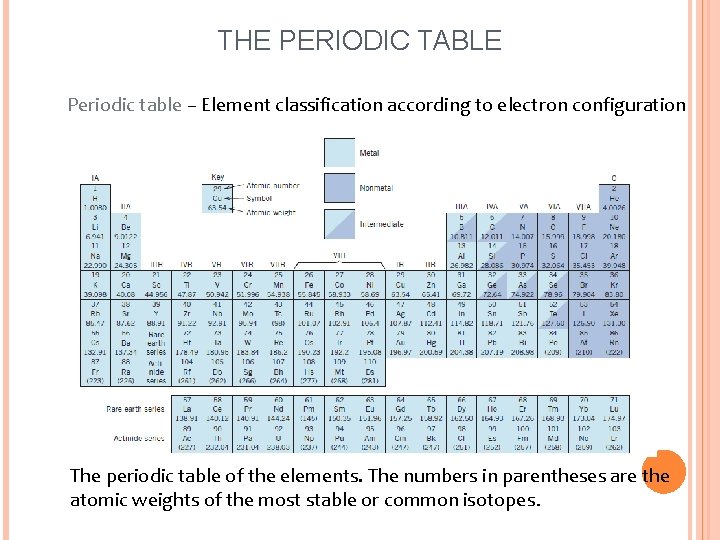
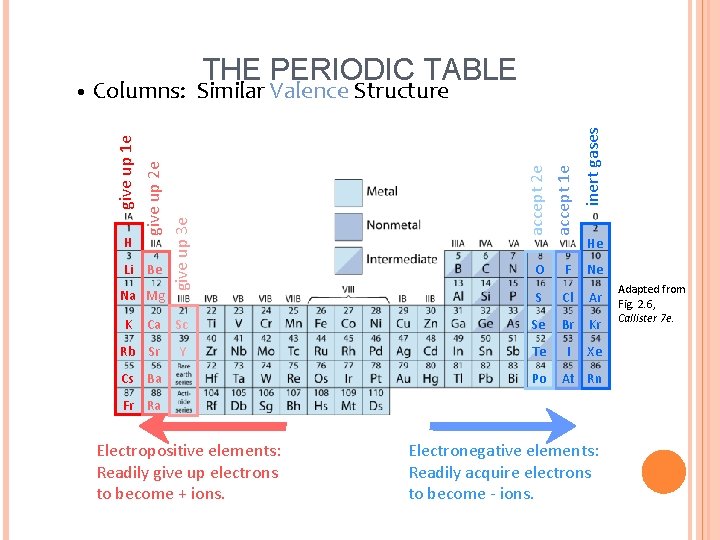
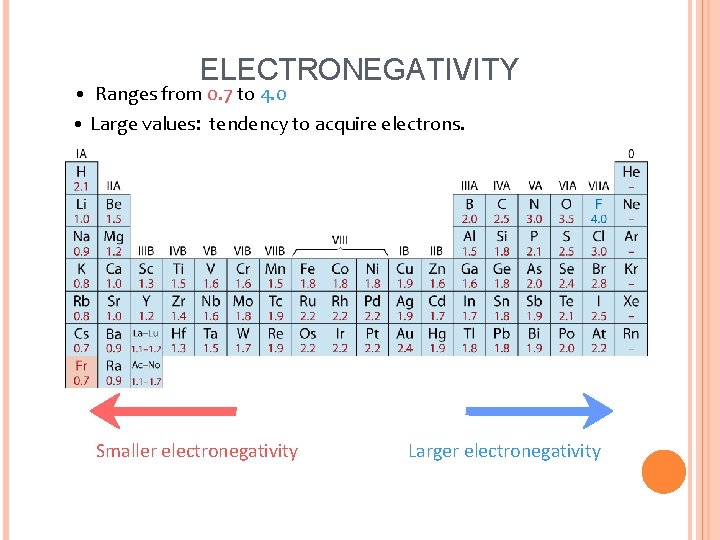
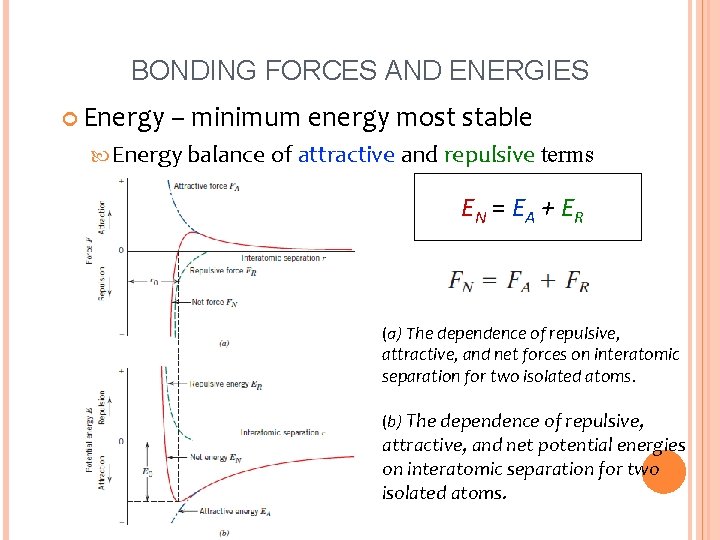
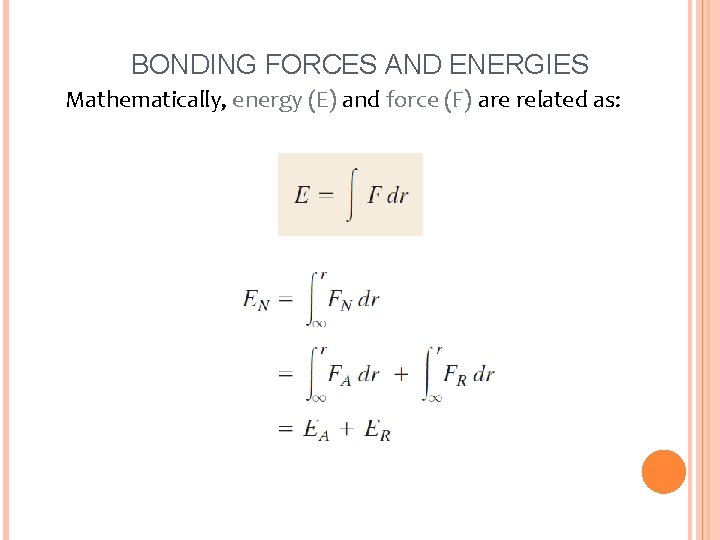
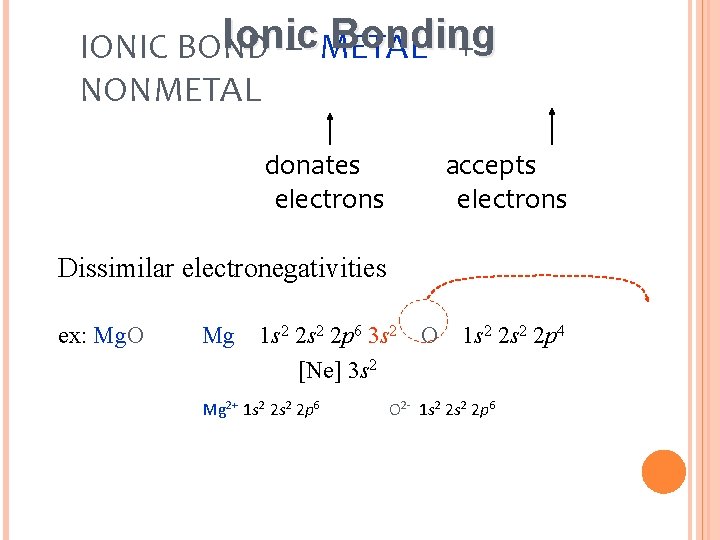
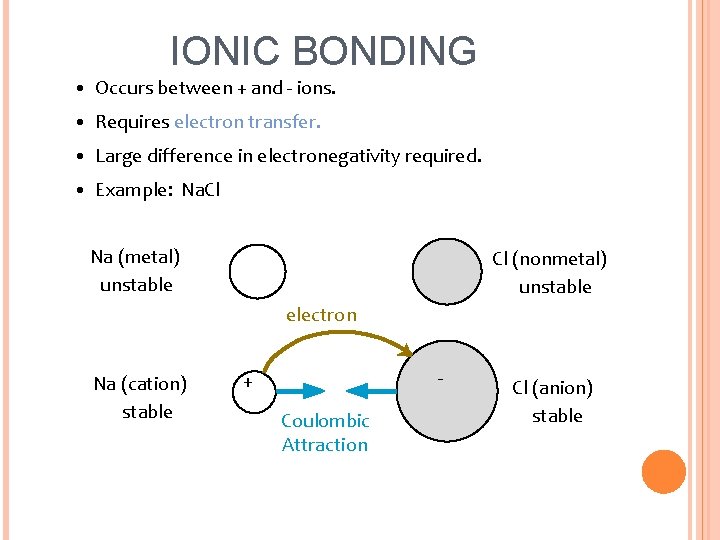
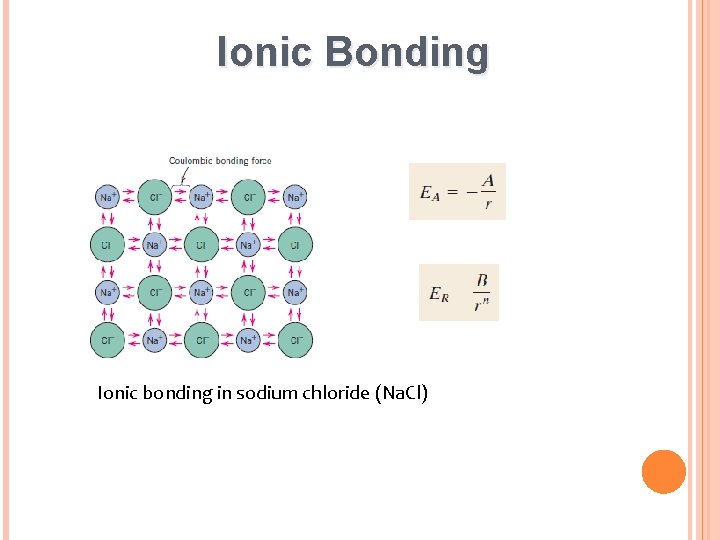
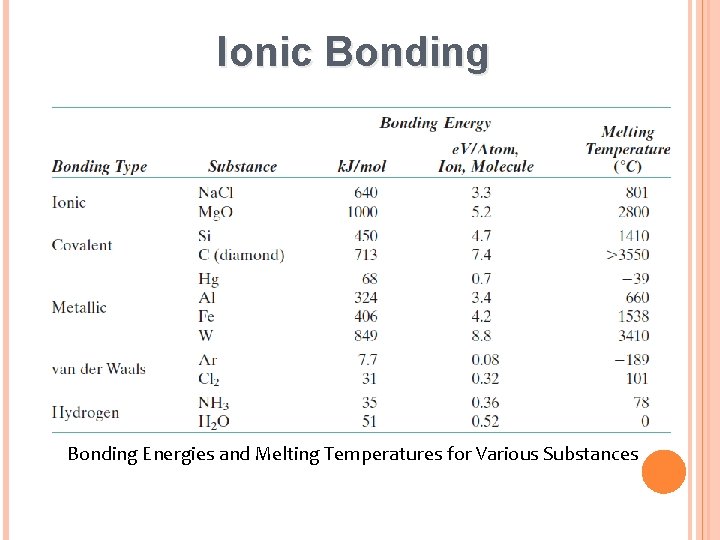
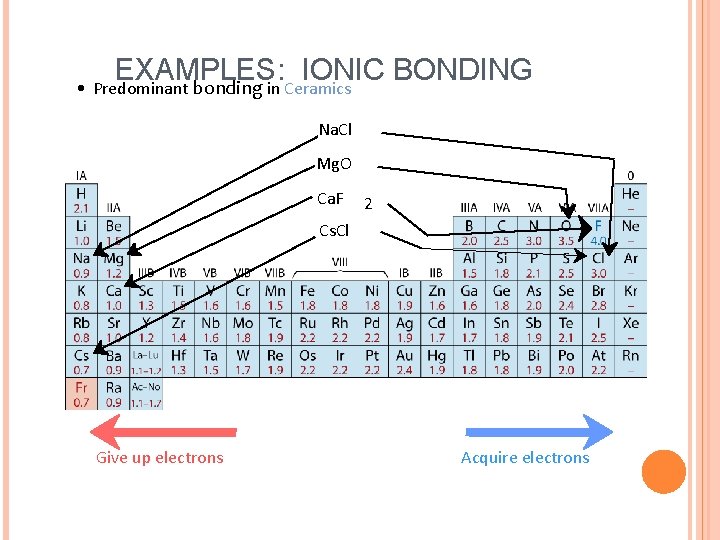
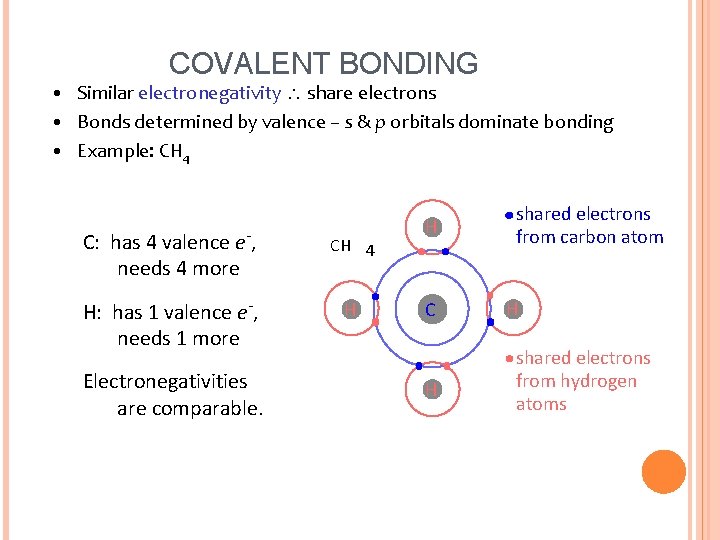
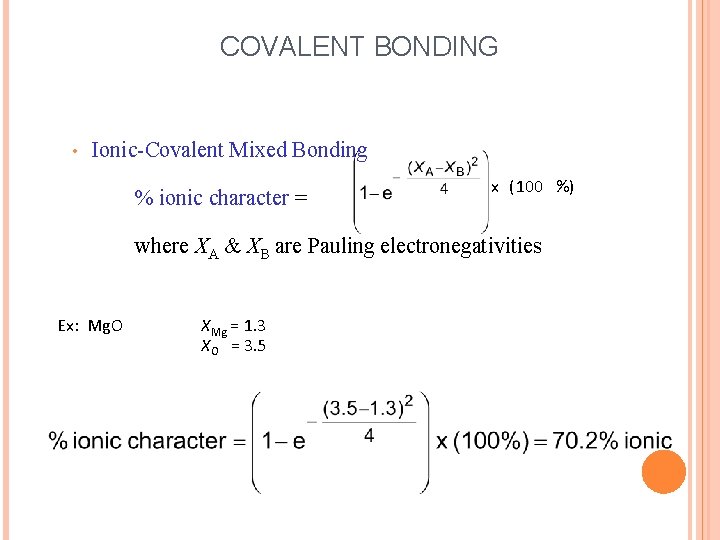
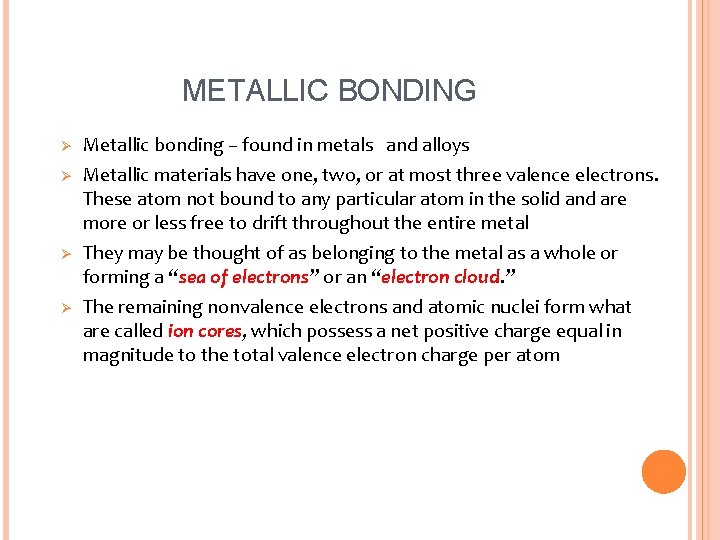
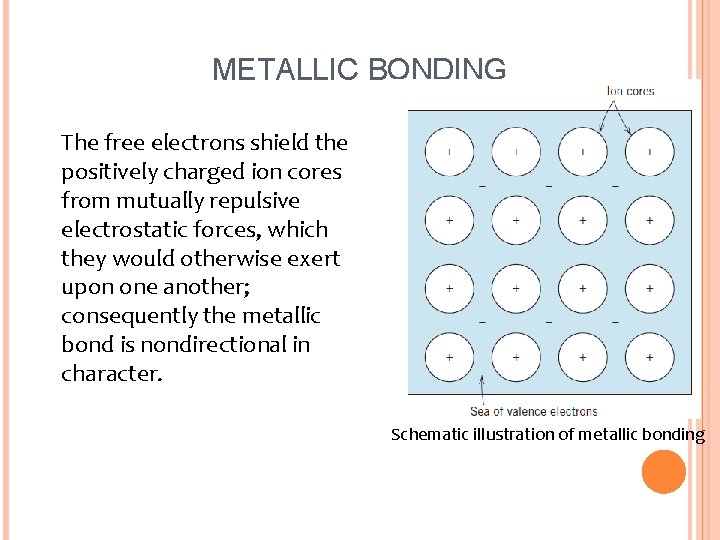
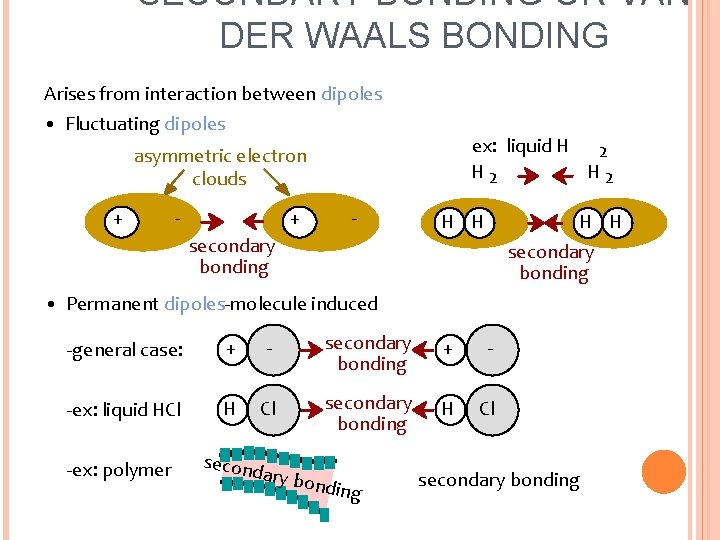
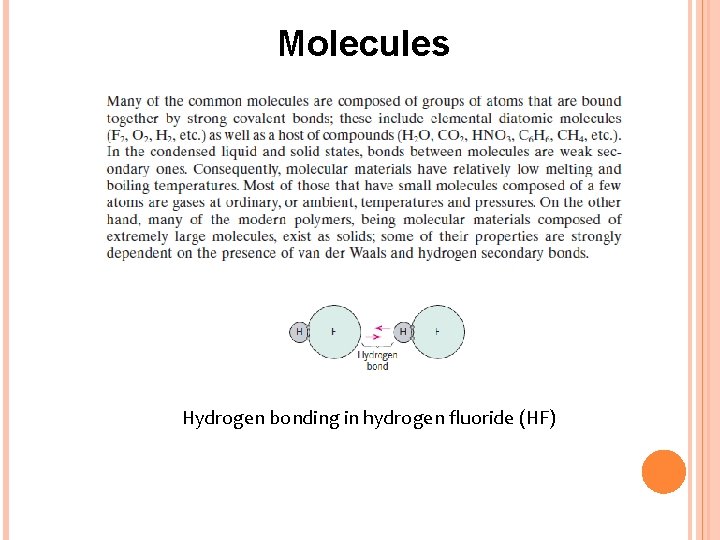
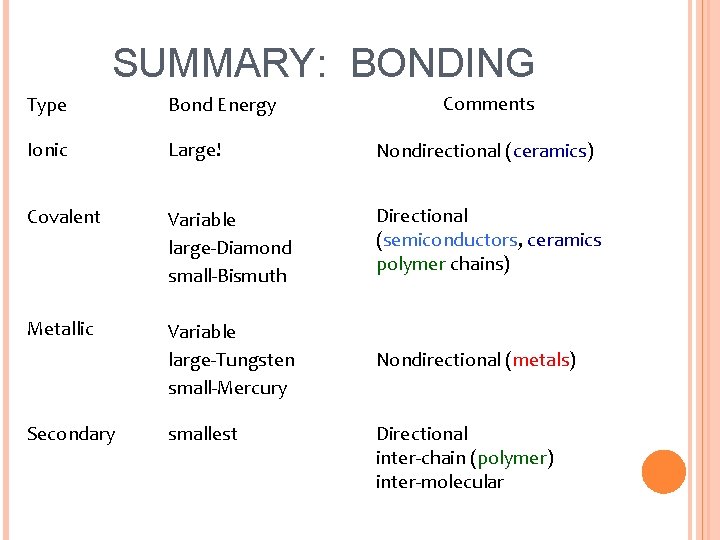
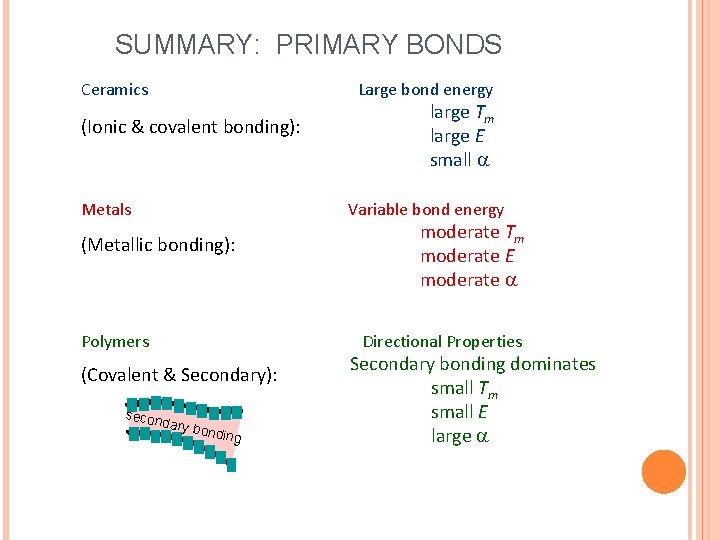
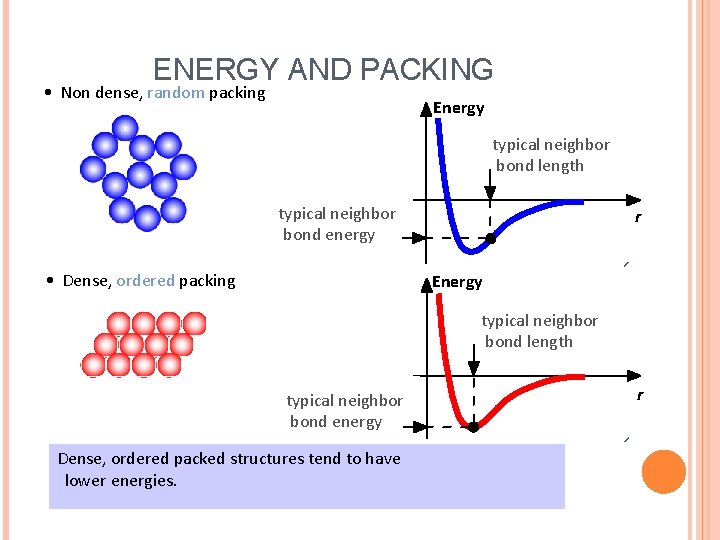
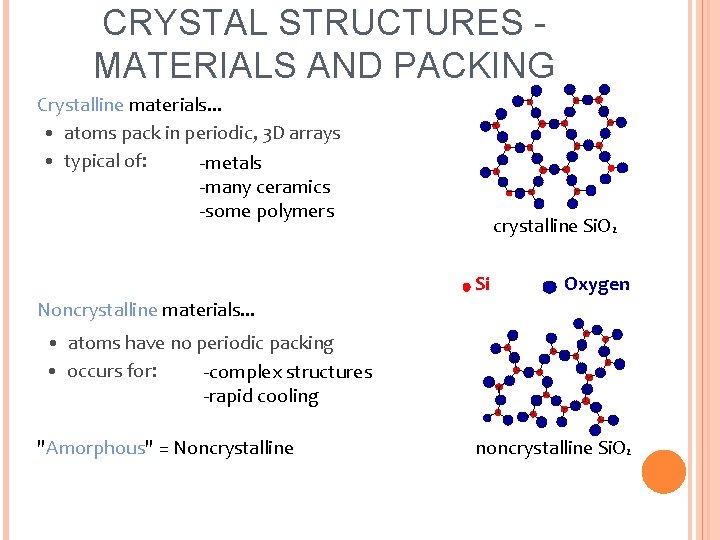
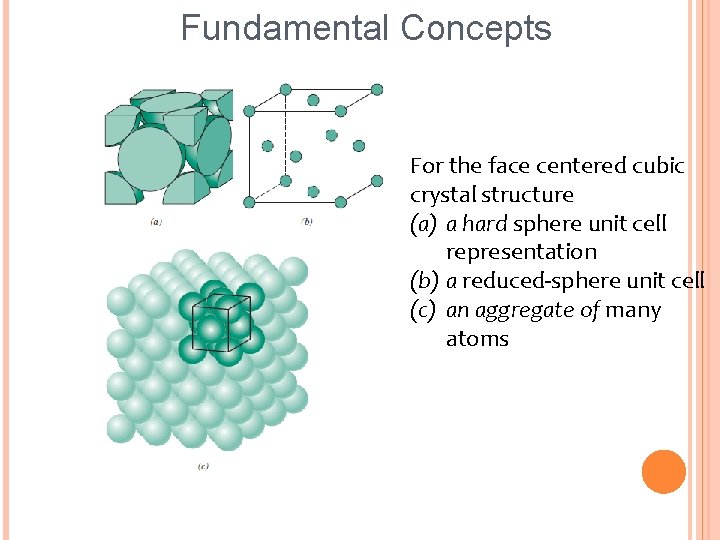
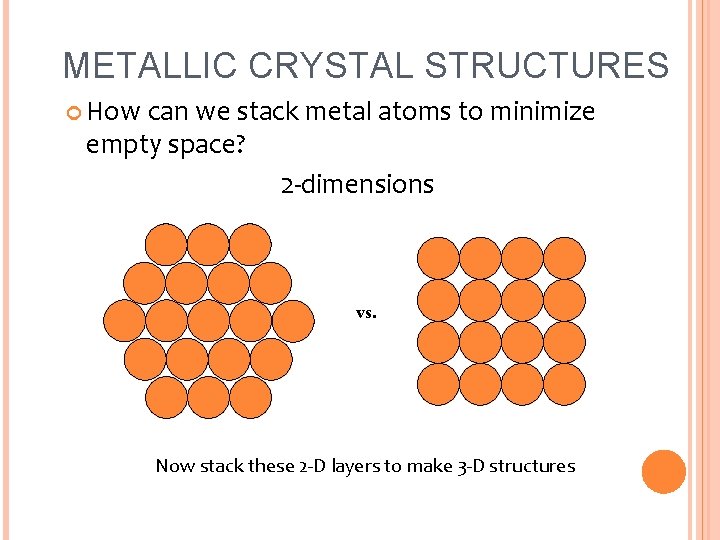
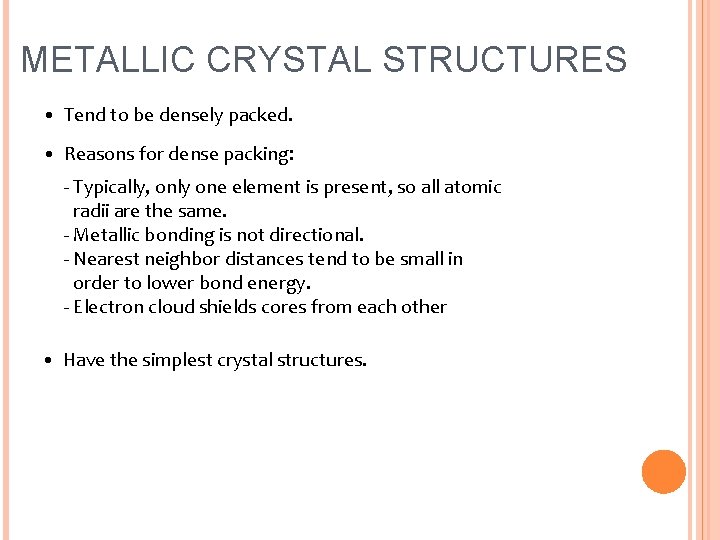
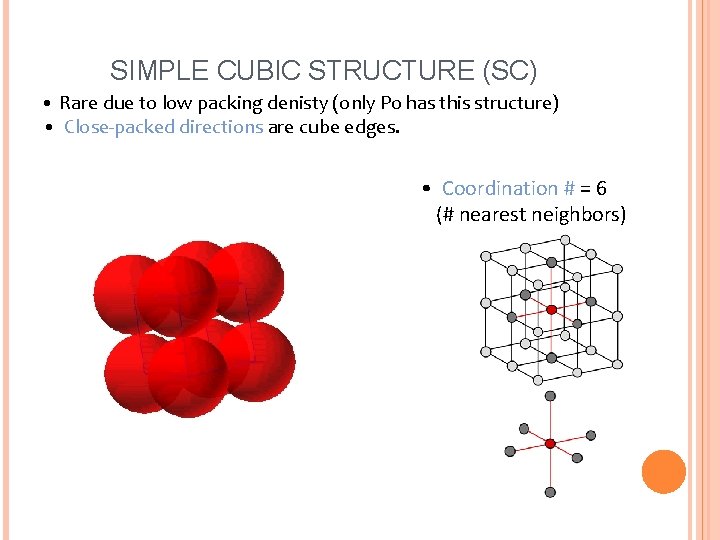
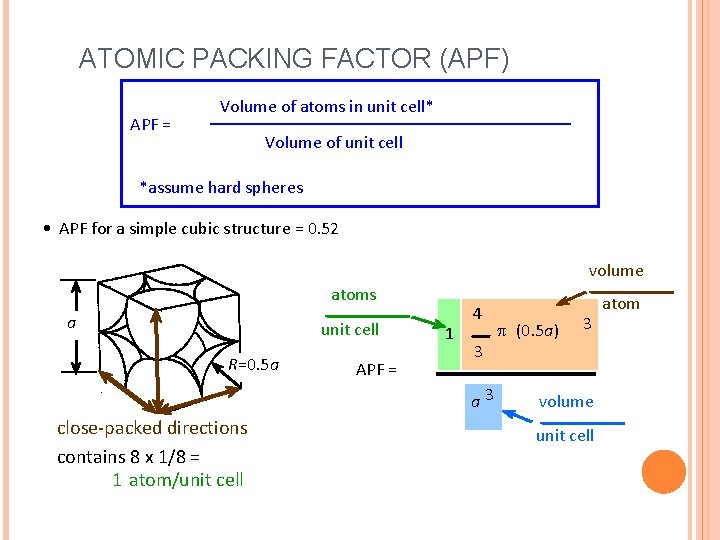
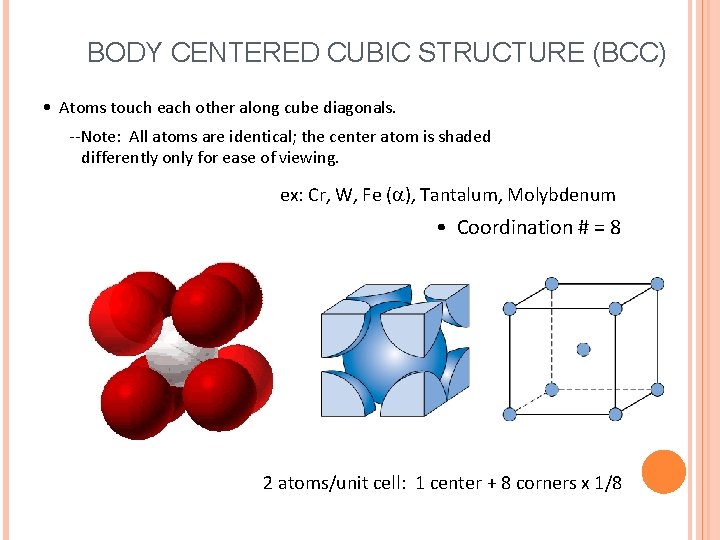
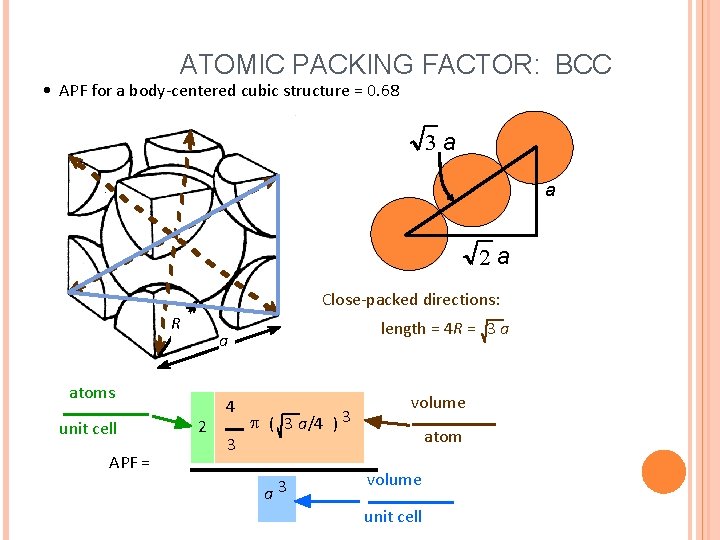
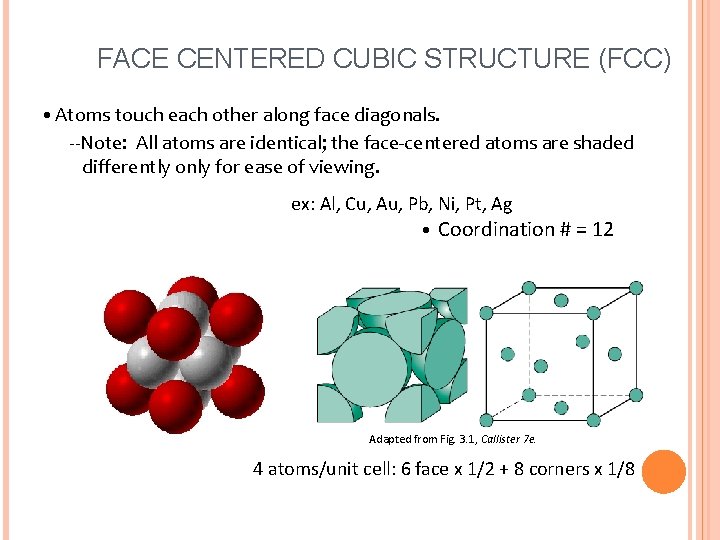
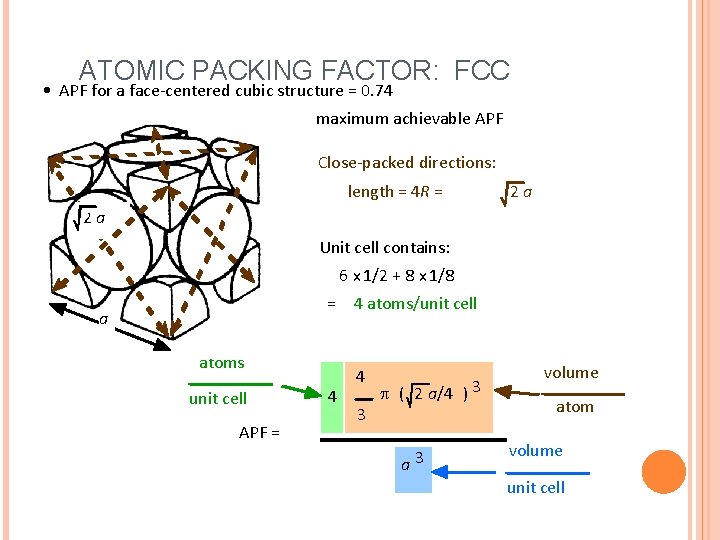
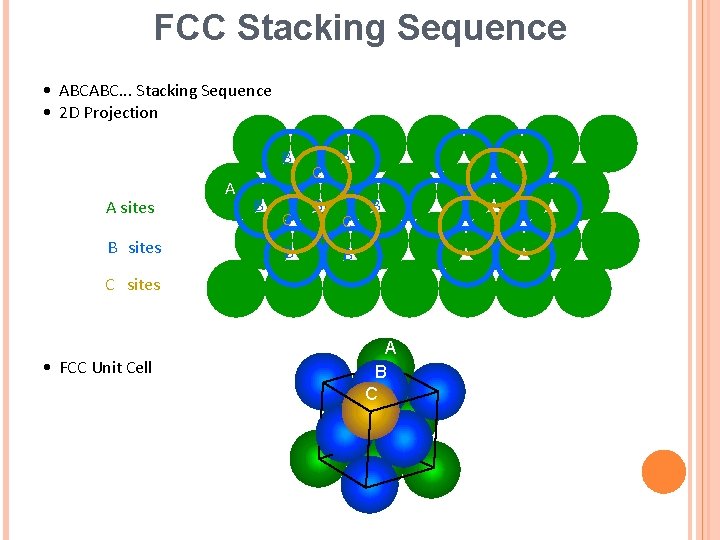
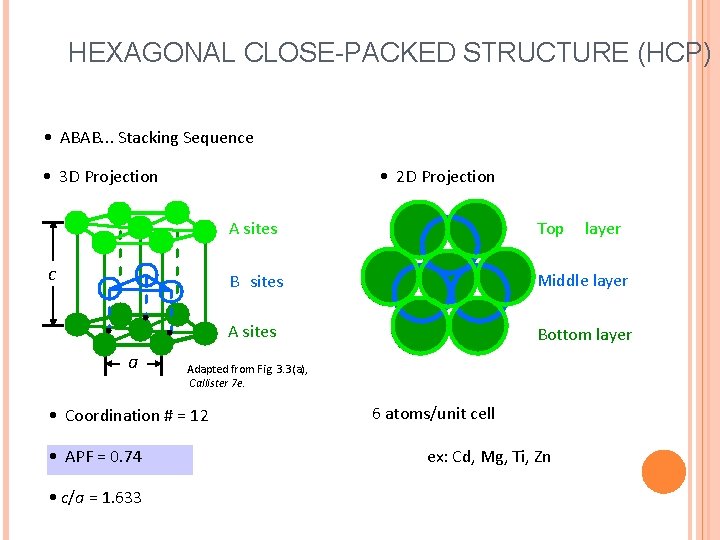
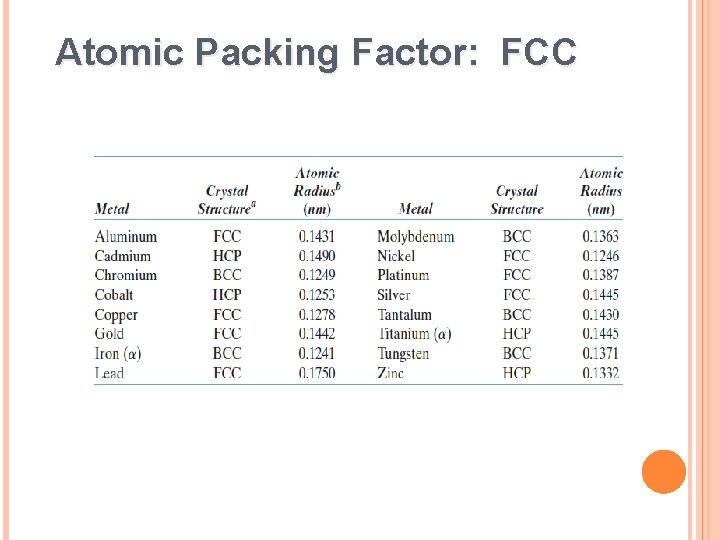
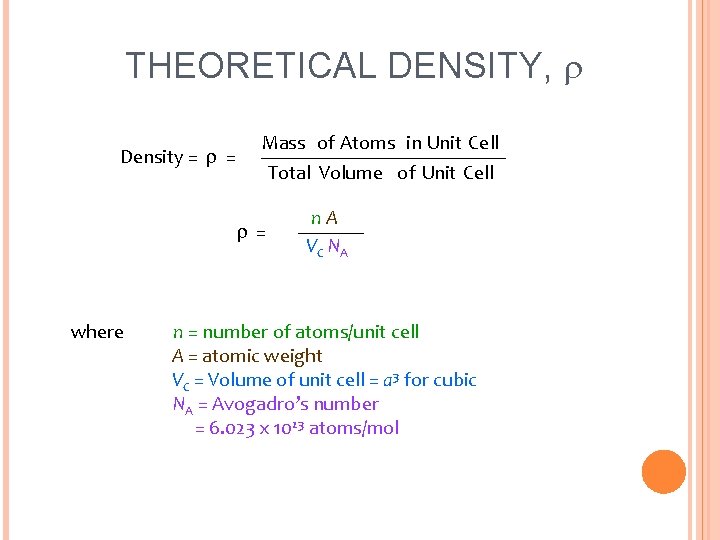
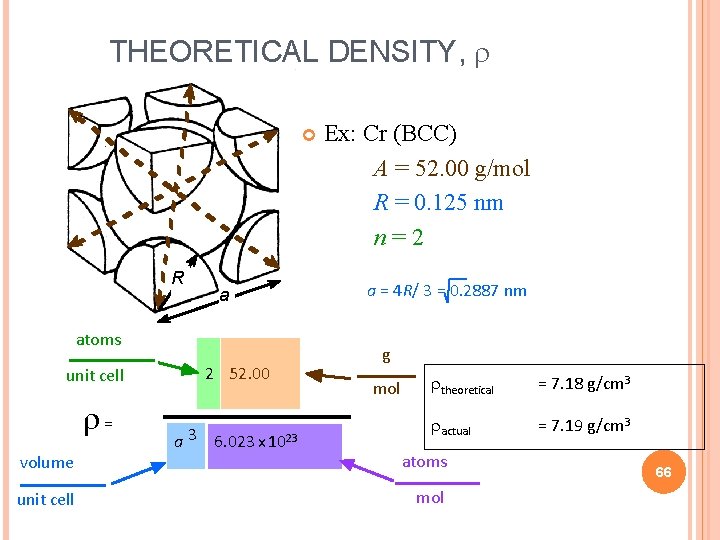
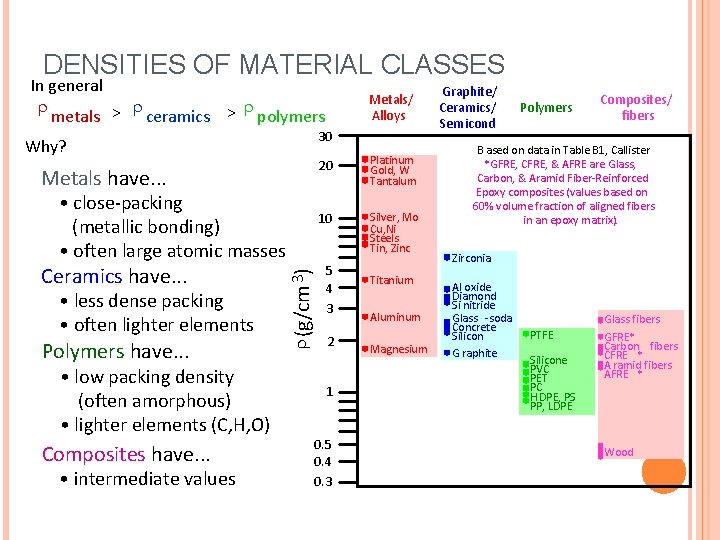
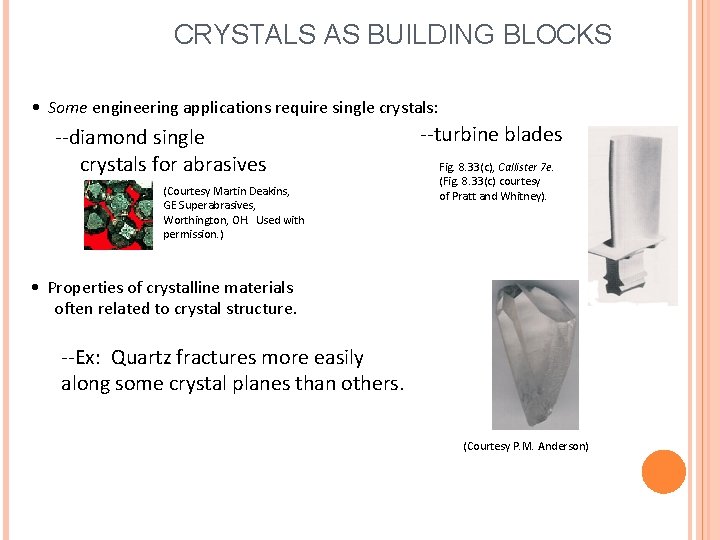
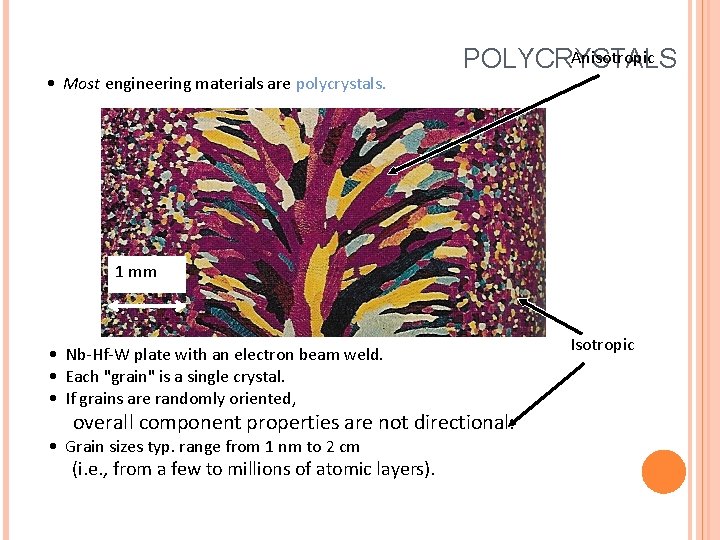
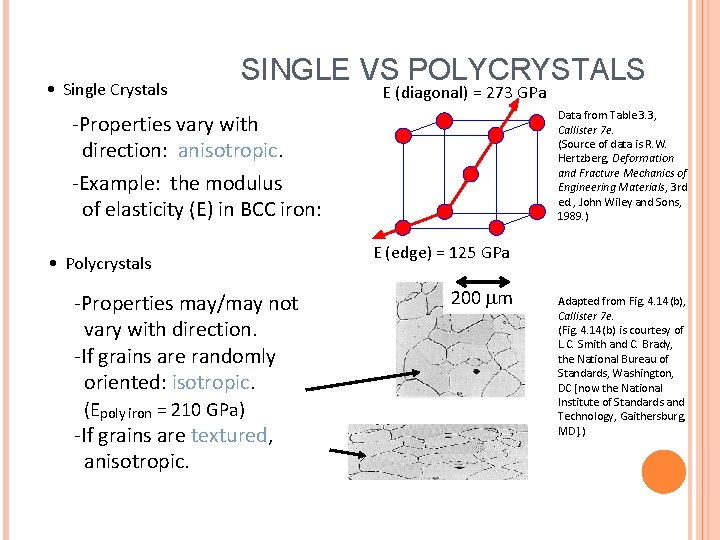
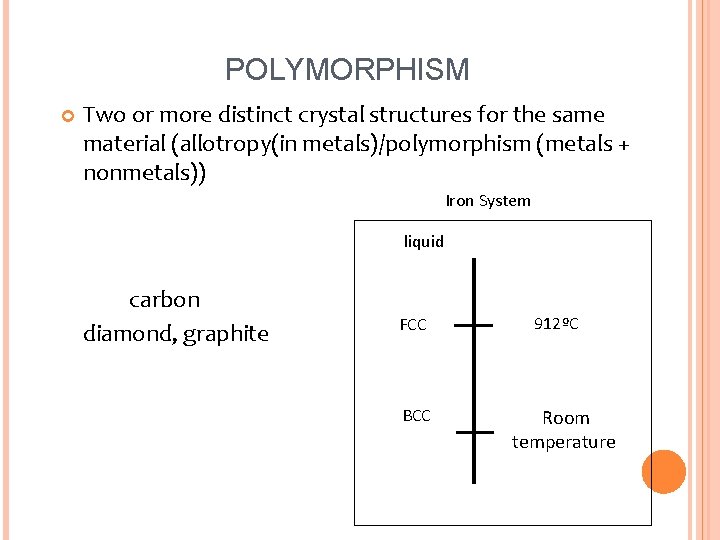
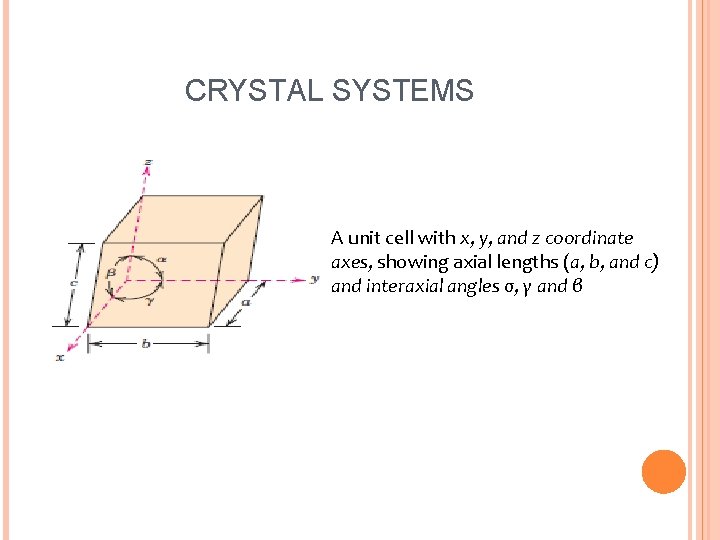
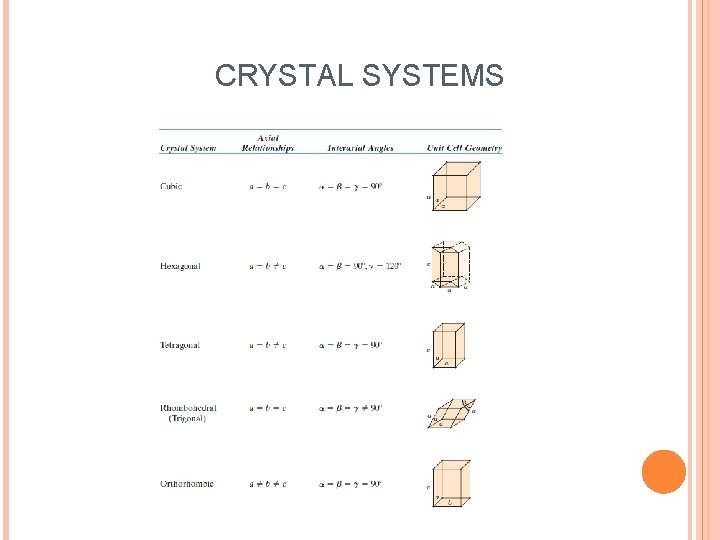
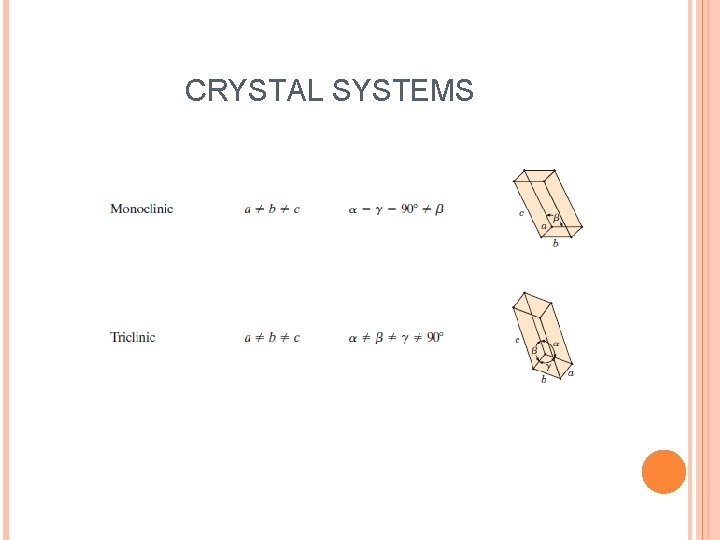
- Slides: 74
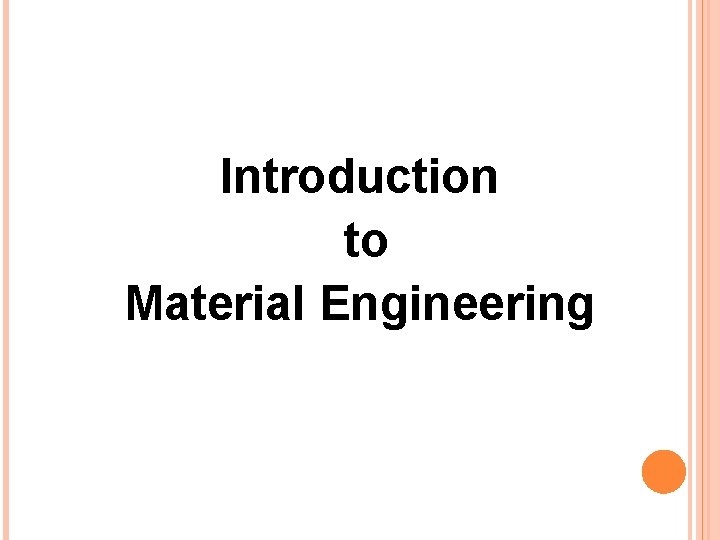
Introduction to Material Engineering
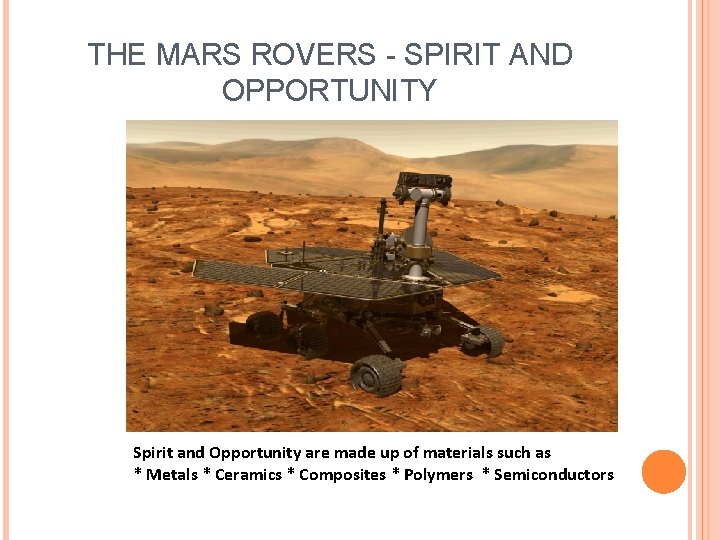
THE MARS ROVERS - SPIRIT AND OPPORTUNITY Spirit and Opportunity are made up of materials such as * Metals * Ceramics * Composites * Polymers * Semiconductors
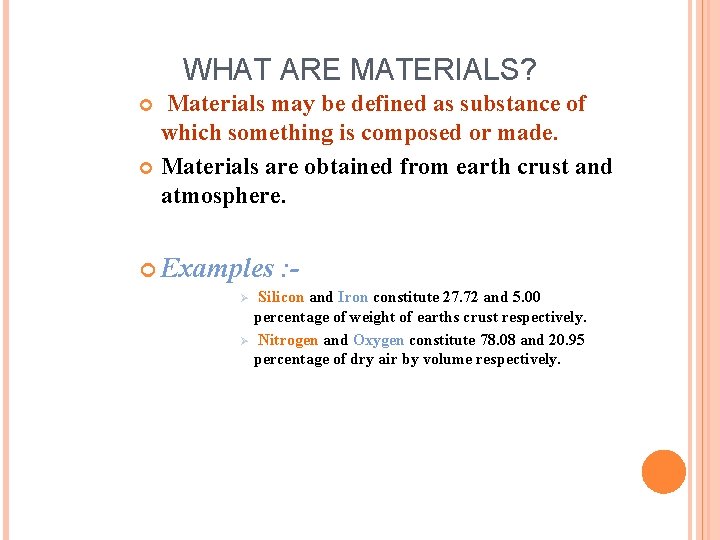
WHAT ARE MATERIALS? Materials may be defined as substance of which something is composed or made. Materials are obtained from earth crust and atmosphere. Examples Ø Ø : - Silicon and Iron constitute 27. 72 and 5. 00 percentage of weight of earths crust respectively. Nitrogen and Oxygen constitute 78. 08 and 20. 95 percentage of dry air by volume respectively.
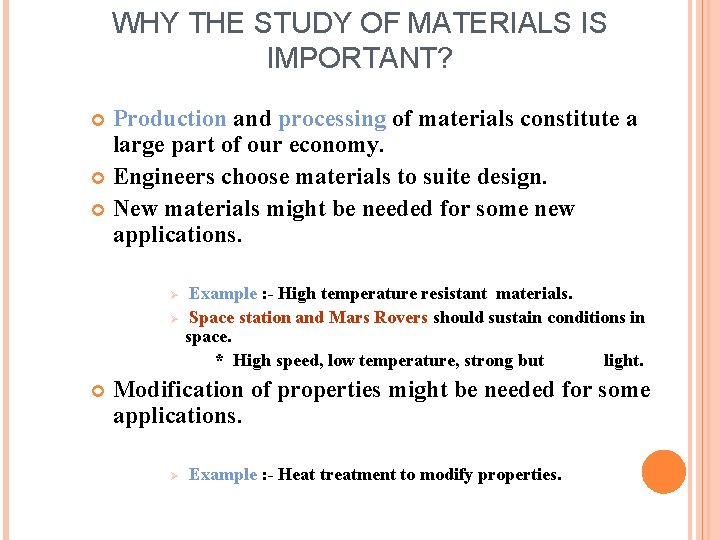
WHY THE STUDY OF MATERIALS IS IMPORTANT? Production and processing of materials constitute a large part of our economy. Engineers choose materials to suite design. New materials might be needed for some new applications. Ø Ø Example : - High temperature resistant materials. Space station and Mars Rovers should sustain conditions in space. * High speed, low temperature, strong but light. Modification of properties might be needed for some applications. Ø Example : - Heat treatment to modify properties.
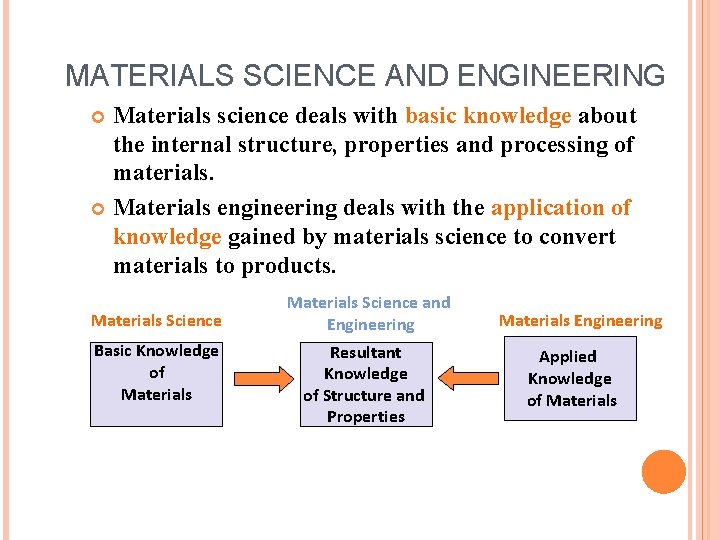
MATERIALS SCIENCE AND ENGINEERING Materials science deals with basic knowledge about the internal structure, properties and processing of materials. Materials engineering deals with the application of knowledge gained by materials science to convert materials to products. Materials Science Basic Knowledge of Materials Science and Engineering Resultant Knowledge of Structure and Properties Materials Engineering Applied Knowledge of Materials
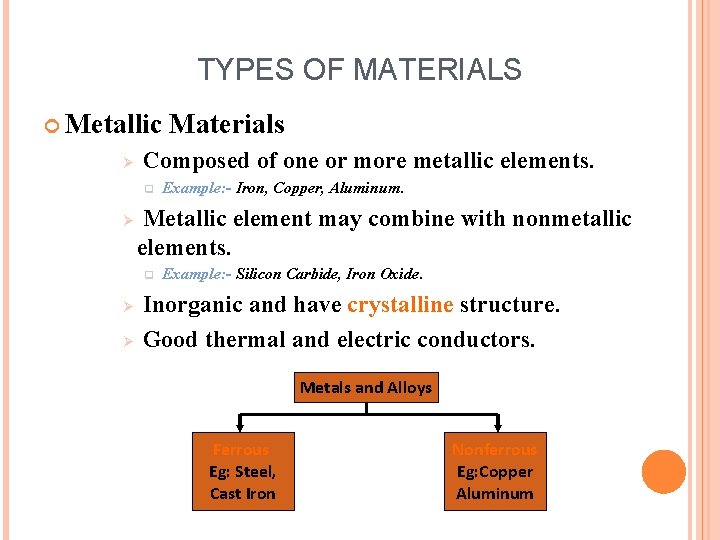
TYPES OF MATERIALS Metallic Ø Composed of one or more metallic elements. q Ø Ø Example: - Iron, Copper, Aluminum. Metallic element may combine with nonmetallic elements. q Ø Materials Example: - Silicon Carbide, Iron Oxide. Inorganic and have crystalline structure. Good thermal and electric conductors. Metals and Alloys Ferrous Eg: Steel, Cast Iron Nonferrous Eg: Copper Aluminum
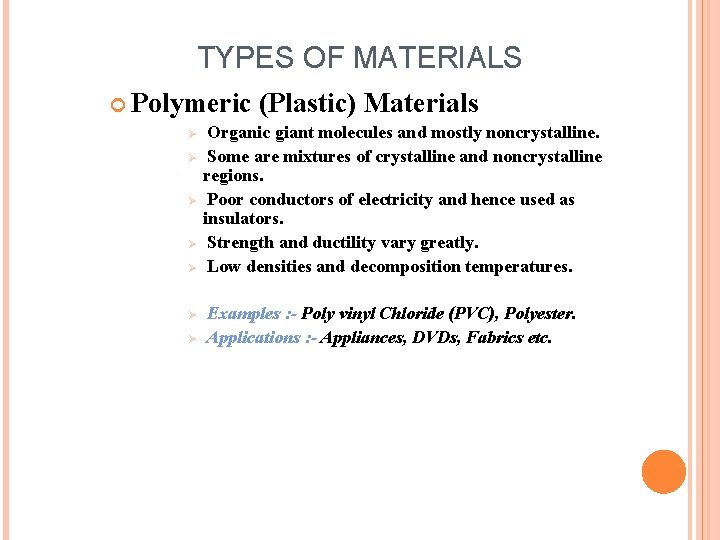
TYPES OF MATERIALS Polymeric Ø Ø Ø Ø (Plastic) Materials Organic giant molecules and mostly noncrystalline. Some are mixtures of crystalline and noncrystalline regions. Poor conductors of electricity and hence used as insulators. Strength and ductility vary greatly. Low densities and decomposition temperatures. Examples : - Poly vinyl Chloride (PVC), Polyester. Applications : - Appliances, DVDs, Fabrics etc.
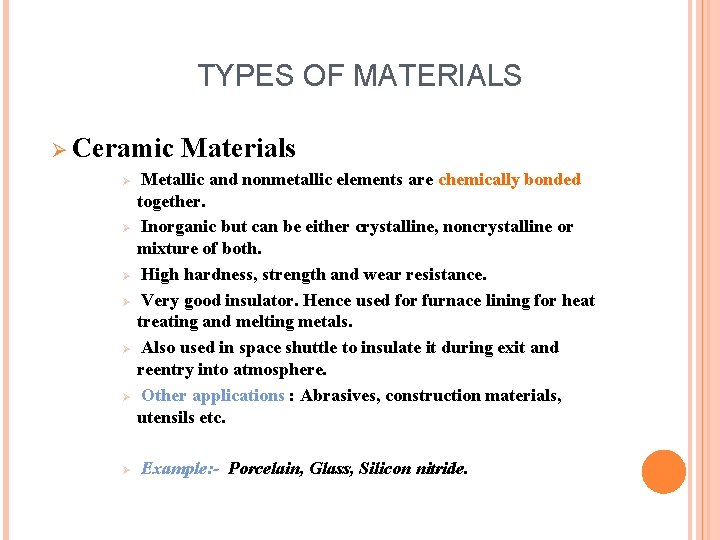
TYPES OF MATERIALS Ø Ceramic Ø Ø Ø Ø Materials Metallic and nonmetallic elements are chemically bonded together. Inorganic but can be either crystalline, noncrystalline or mixture of both. High hardness, strength and wear resistance. Very good insulator. Hence used for furnace lining for heat treating and melting metals. Also used in space shuttle to insulate it during exit and reentry into atmosphere. Other applications : Abrasives, construction materials, utensils etc. Example: - Porcelain, Glass, Silicon nitride.
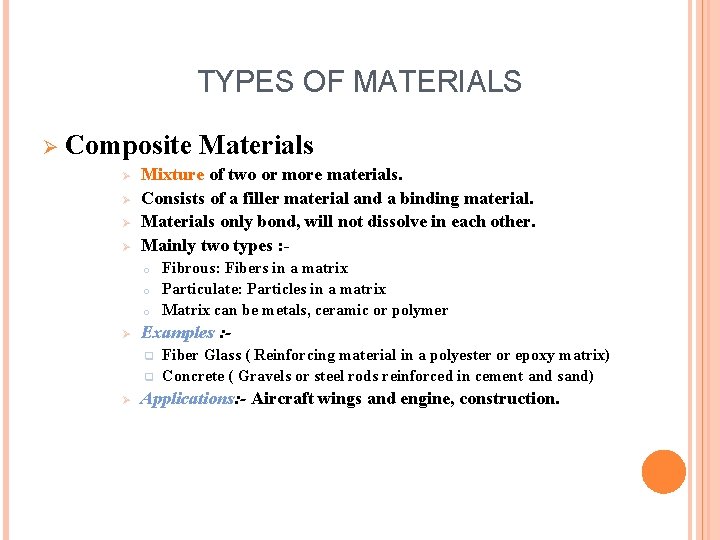
TYPES OF MATERIALS Ø Composite Ø Ø Mixture of two or more materials. Consists of a filler material and a binding material. Materials only bond, will not dissolve in each other. Mainly two types : o o o Ø Fibrous: Fibers in a matrix Particulate: Particles in a matrix Matrix can be metals, ceramic or polymer Examples : q q Ø Materials Fiber Glass ( Reinforcing material in a polyester or epoxy matrix) Concrete ( Gravels or steel rods reinforced in cement and sand) Applications: - Aircraft wings and engine, construction.
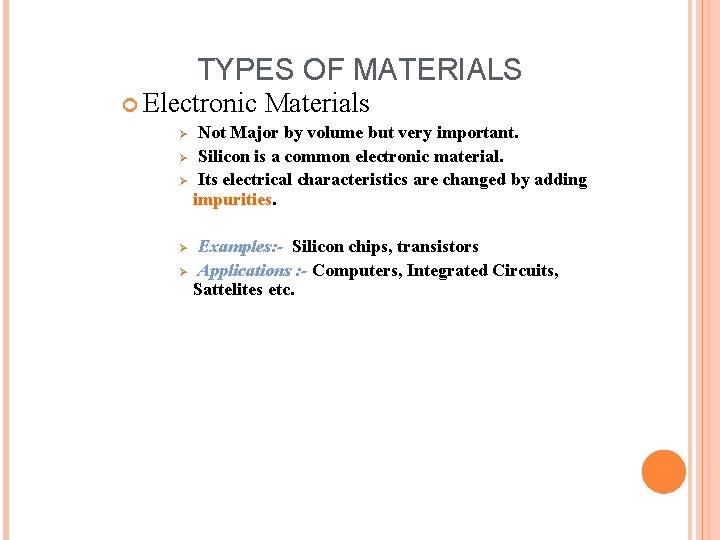
TYPES OF MATERIALS Electronic Ø Ø Ø Materials Not Major by volume but very important. Silicon is a common electronic material. Its electrical characteristics are changed by adding impurities. Examples: - Silicon chips, transistors Applications : - Computers, Integrated Circuits, Sattelites etc.
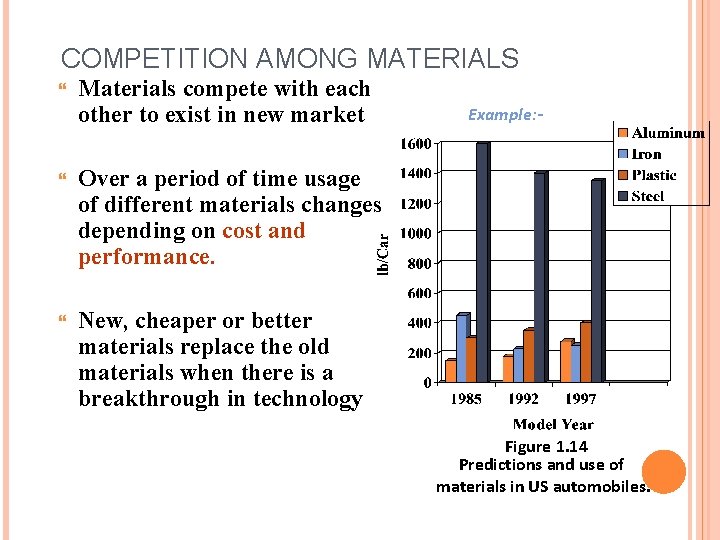
COMPETITION AMONG MATERIALS Materials compete with each other to exist in new market Over a period of time usage of different materials changes depending on cost and performance. New, cheaper or better materials replace the old materials when there is a breakthrough in technology Example: - Figure 1. 14 Predictions and use of materials in US automobiles.
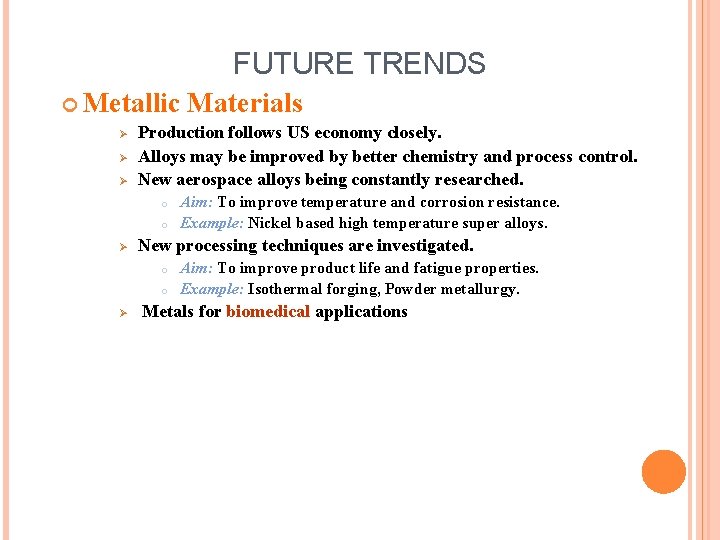
FUTURE TRENDS Metallic Ø Ø Ø Production follows US economy closely. Alloys may be improved by better chemistry and process control. New aerospace alloys being constantly researched. o o Ø Aim: To improve temperature and corrosion resistance. Example: Nickel based high temperature super alloys. New processing techniques are investigated. o o Ø Materials Aim: To improve product life and fatigue properties. Example: Isothermal forging, Powder metallurgy. Metals for biomedical applications
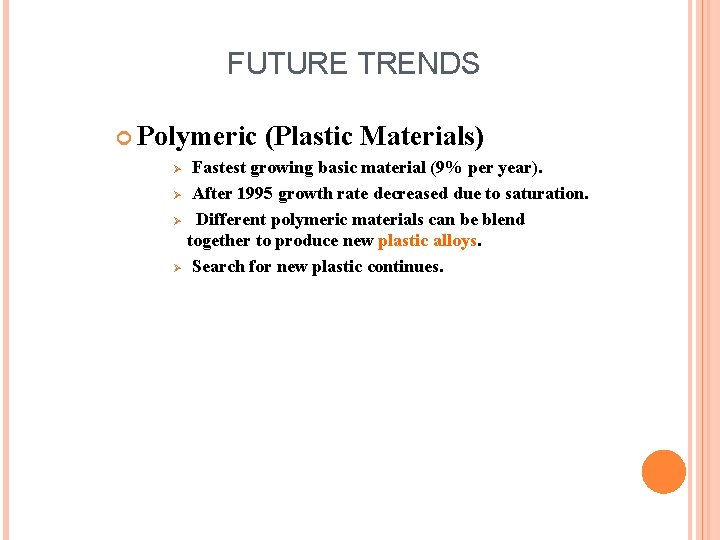
FUTURE TRENDS Polymeric Ø Ø (Plastic Materials) Fastest growing basic material (9% per year). After 1995 growth rate decreased due to saturation. Different polymeric materials can be blend together to produce new plastic alloys. Search for new plastic continues.
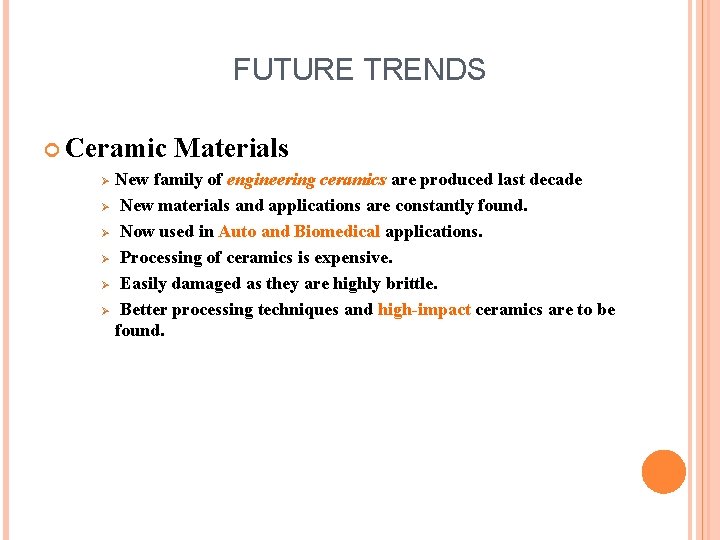
FUTURE TRENDS Ceramic Ø Ø Ø Materials New family of engineering ceramics are produced last decade New materials and applications are constantly found. Now used in Auto and Biomedical applications. Processing of ceramics is expensive. Easily damaged as they are highly brittle. Better processing techniques and high-impact ceramics are to be found.
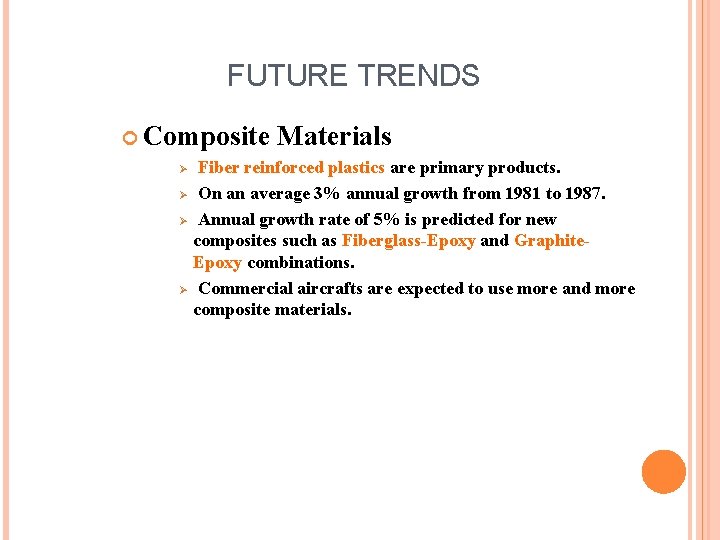
FUTURE TRENDS Composite Ø Ø Materials Fiber reinforced plastics are primary products. On an average 3% annual growth from 1981 to 1987. Annual growth rate of 5% is predicted for new composites such as Fiberglass-Epoxy and Graphite. Epoxy combinations. Commercial aircrafts are expected to use more and more composite materials.
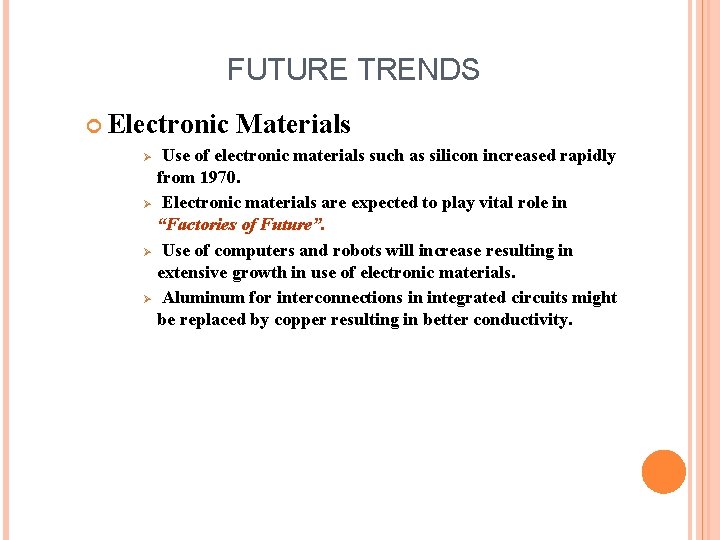
FUTURE TRENDS Electronic Ø Ø Materials Use of electronic materials such as silicon increased rapidly from 1970. Electronic materials are expected to play vital role in “Factories of Future”. Use of computers and robots will increase resulting in extensive growth in use of electronic materials. Aluminum for interconnections in integrated circuits might be replaced by copper resulting in better conductivity.
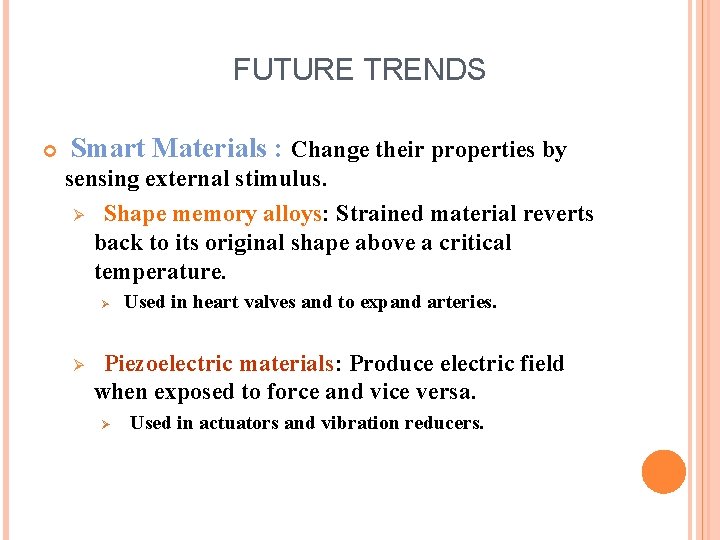
FUTURE TRENDS Smart Materials : Change their properties by sensing external stimulus. Ø Shape memory alloys: Strained material reverts back to its original shape above a critical temperature. Ø Ø Used in heart valves and to expand arteries. Piezoelectric materials: Produce electric field when exposed to force and vice versa. Ø Used in actuators and vibration reducers.
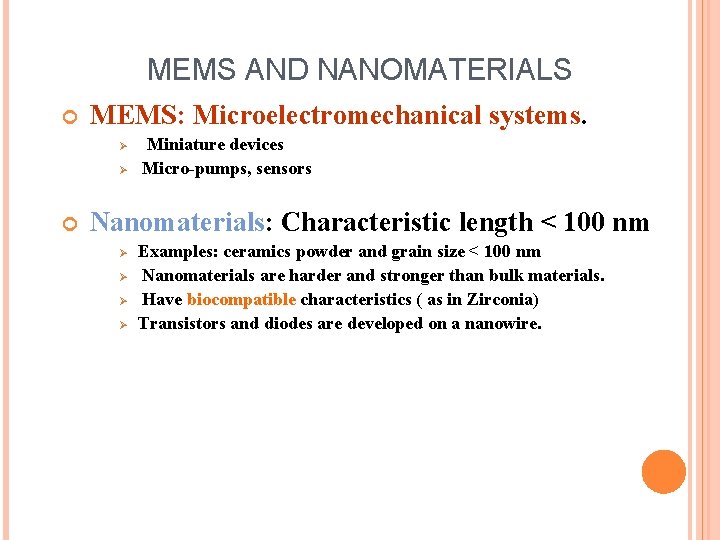
MEMS AND NANOMATERIALS MEMS: Microelectromechanical systems. Ø Ø Miniature devices Micro-pumps, sensors Nanomaterials: Characteristic length < 100 nm Ø Ø Examples: ceramics powder and grain size < 100 nm Nanomaterials are harder and stronger than bulk materials. Have biocompatible characteristics ( as in Zirconia) Transistors and diodes are developed on a nanowire.
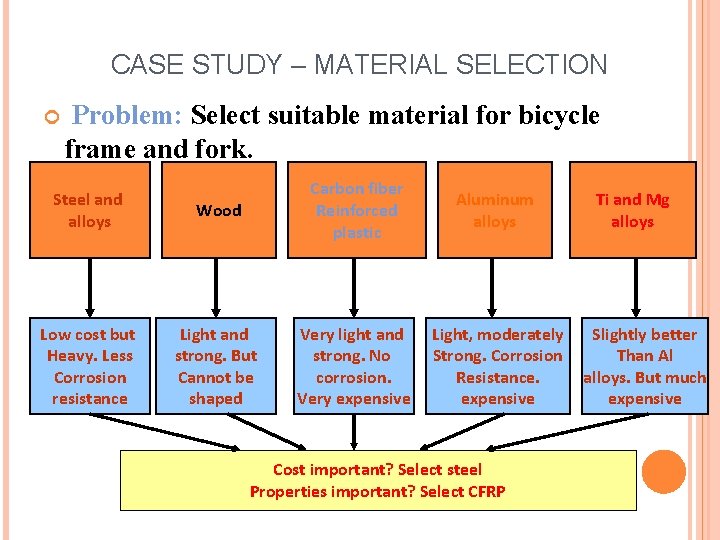
CASE STUDY – MATERIAL SELECTION Problem: Select suitable material for bicycle frame and fork. Steel and alloys Low cost but Heavy. Less Corrosion resistance Wood Carbon fiber Reinforced plastic Aluminum alloys Light and strong. But Cannot be shaped Very light and strong. No corrosion. Very expensive Light, moderately Strong. Corrosion Resistance. expensive Cost important? Select steel Properties important? Select CFRP Ti and Mg alloys Slightly better Than Al alloys. But much expensive
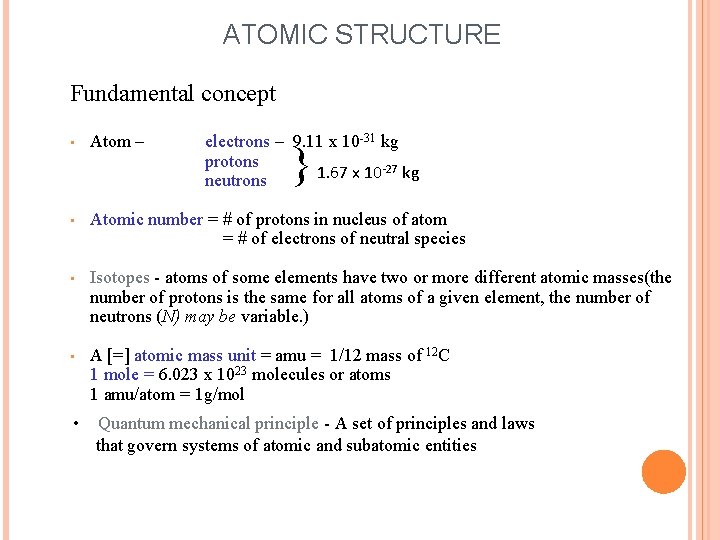
ATOMIC STRUCTURE Fundamental concept • Atom – electrons – 9. 11 x 10 -31 kg protons 1. 67 x 10 -27 kg neutrons • Atomic number = # of protons in nucleus of atom = # of electrons of neutral species • Isotopes - atoms of some elements have two or more different atomic masses(the number of protons is the same for all atoms of a given element, the number of neutrons (N) may be variable. ) • A [=] atomic mass unit = amu = 1/12 mass of 12 C 1 mole = 6. 023 x 1023 molecules or atoms 1 amu/atom = 1 g/mol } • Quantum mechanical principle - A set of principles and laws that govern systems of atomic and subatomic entities
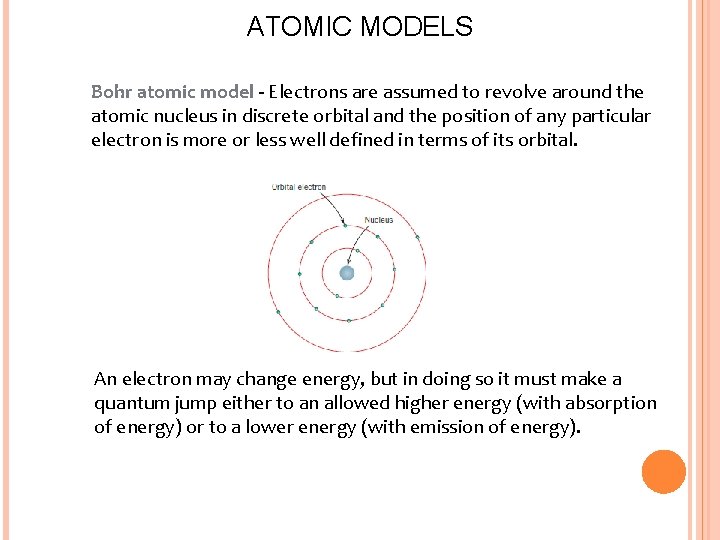
ATOMIC MODELS Bohr atomic model - Electrons are assumed to revolve around the atomic nucleus in discrete orbital and the position of any particular electron is more or less well defined in terms of its orbital. An electron may change energy, but in doing so it must make a quantum jump either to an allowed higher energy (with absorption of energy) or to a lower energy (with emission of energy).
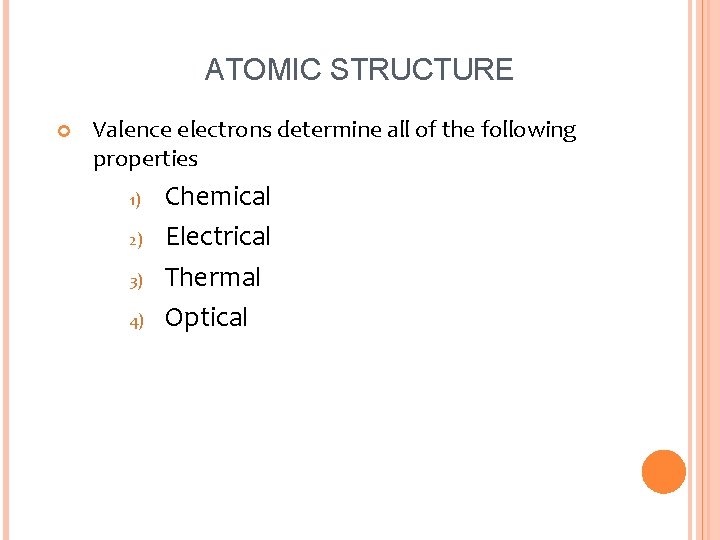
ATOMIC STRUCTURE Valence electrons determine all of the following properties 1) 2) 3) 4) Chemical Electrical Thermal Optical
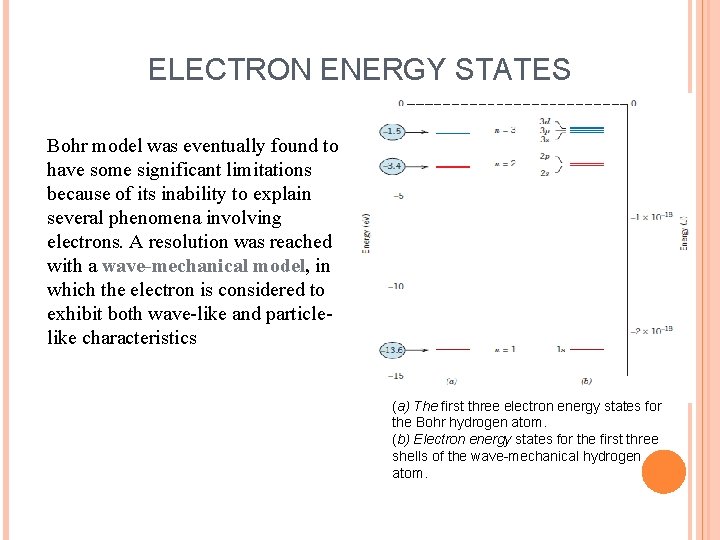
ELECTRON ENERGY STATES Bohr model was eventually found to have some significant limitations because of its inability to explain several phenomena involving electrons. A resolution was reached with a wave-mechanical model, in which the electron is considered to exhibit both wave-like and particlelike characteristics (a) The first three electron energy states for the Bohr hydrogen atom. (b) Electron energy states for the first three shells of the wave-mechanical hydrogen atom.
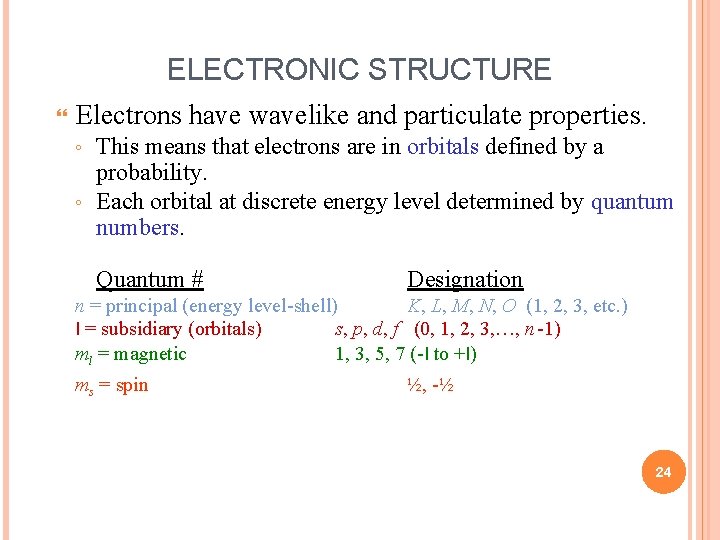
ELECTRONIC STRUCTURE Electrons have wavelike and particulate properties. This means that electrons are in orbitals defined by a probability. ◦ Each orbital at discrete energy level determined by quantum numbers. ◦ Quantum # Designation n = principal (energy level-shell) K, L, M, N, O (1, 2, 3, etc. ) l = subsidiary (orbitals) s, p, d, f (0, 1, 2, 3, …, n -1) ml = magnetic 1, 3, 5, 7 (-l to +l) ms = spin ½, -½ 24
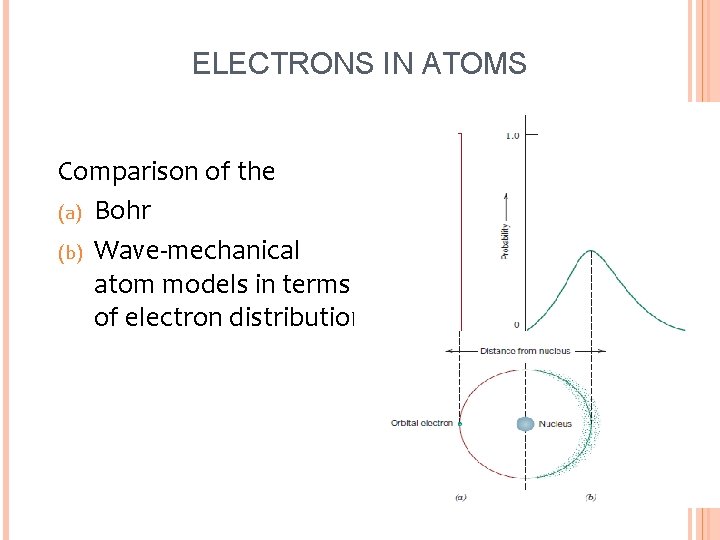
ELECTRONS IN ATOMS Comparison of the (a) Bohr (b) Wave-mechanical atom models in terms of electron distribution
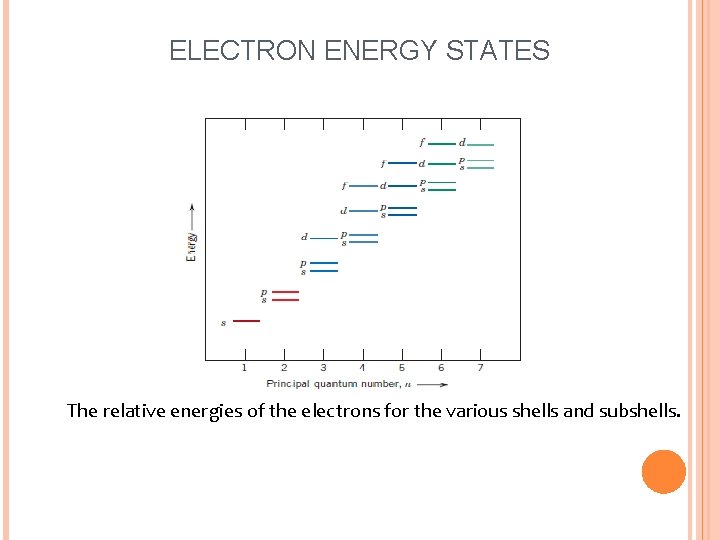
ELECTRON ENERGY STATES The relative energies of the electrons for the various shells and subshells.
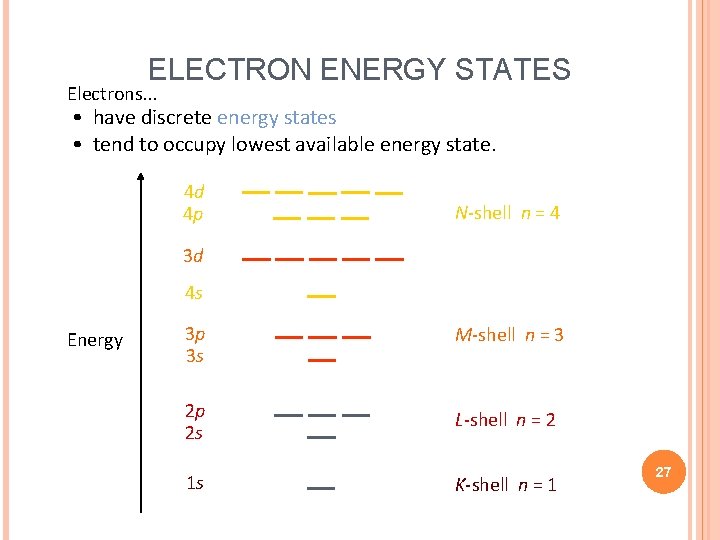
ELECTRON ENERGY STATES Electrons. . . • have discrete energy states • tend to occupy lowest available energy state. 4 d 4 p N-shell n = 4 3 d 4 s Energy 3 p 3 s M-shell n = 3 2 p 2 s L-shell n = 2 1 s K-shell n = 1 27
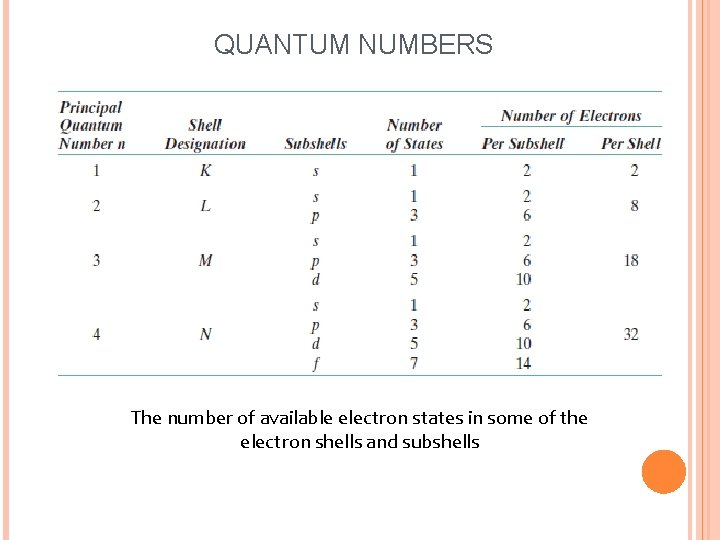
QUANTUM NUMBERS The number of available electron states in some of the electron shells and subshells
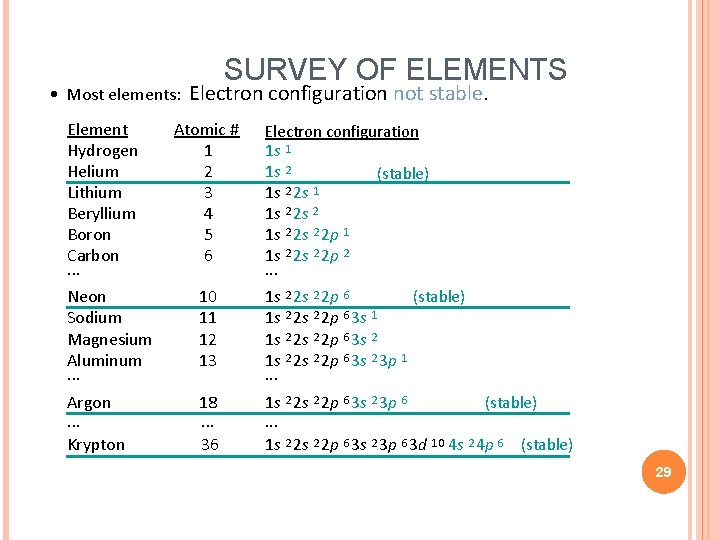
• Most elements: Element Hydrogen Helium Lithium Beryllium Boron Carbon. . . Neon Sodium Magnesium Aluminum. . . Argon. . . Krypton SURVEY OF ELEMENTS Electron configuration not stable. Atomic # 1 2 3 4 5 6 Electron configuration 1 s 1 1 s 2 (stable) 1 s 2 2 s 1 1 s 2 2 s 2 2 p 1 1 s 2 2 p 2. . . 10 11 12 13 1 s 2 2 p 6 (stable) 1 s 2 2 p 6 3 s 1 1 s 2 2 s 2 2 p 6 3 s 2 3 p 1. . . 18. . . 36 1 s 2 2 p 6 3 s 2 3 p 6 (stable). . . 1 s 2 2 p 6 3 s 2 3 p 6 3 d 10 4 s 2 4 p 6 (stable) 29
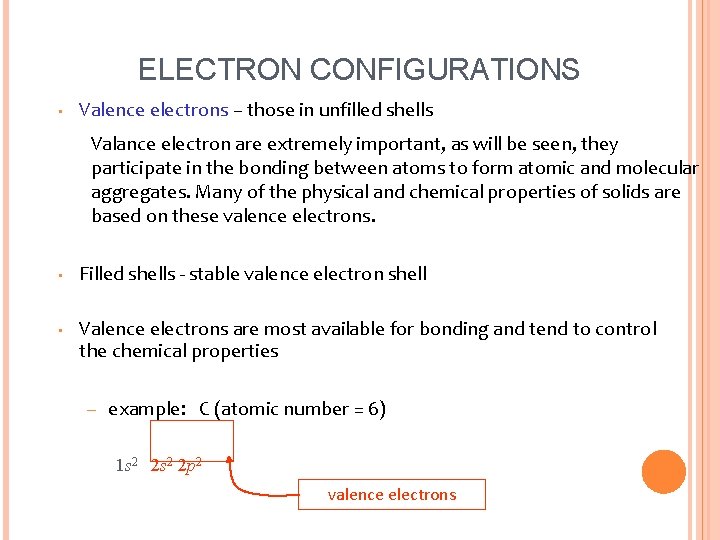
ELECTRON CONFIGURATIONS • Valence electrons – those in unfilled shells Valance electron are extremely important, as will be seen, they participate in the bonding between atoms to form atomic and molecular aggregates. Many of the physical and chemical properties of solids are based on these valence electrons. • Filled shells - stable valence electron shell • Valence electrons are most available for bonding and tend to control the chemical properties – example: C (atomic number = 6) 1 s 2 2 p 2 valence electrons
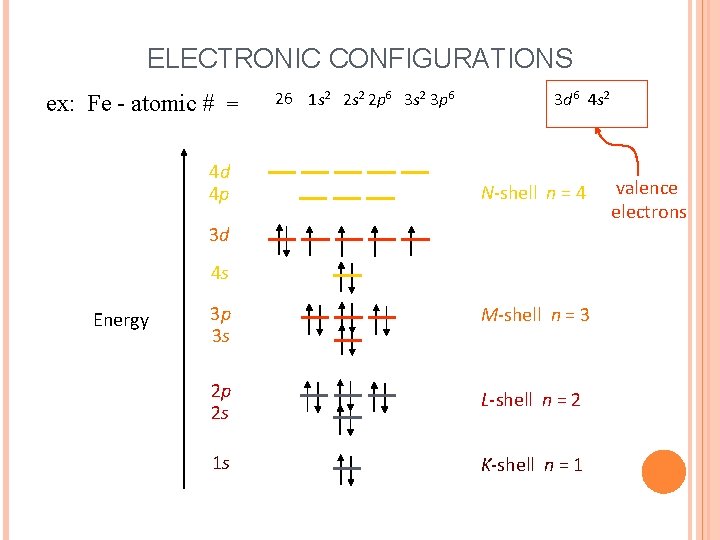
ELECTRONIC CONFIGURATIONS ex: Fe - atomic # = 4 d 4 p 26 1 s 2 2 p 6 3 s 2 3 p 6 3 d 6 4 s 2 N-shell n = 4 3 d 4 s Energy 3 p 3 s M-shell n = 3 2 p 2 s L-shell n = 2 1 s K-shell n = 1 valence electrons
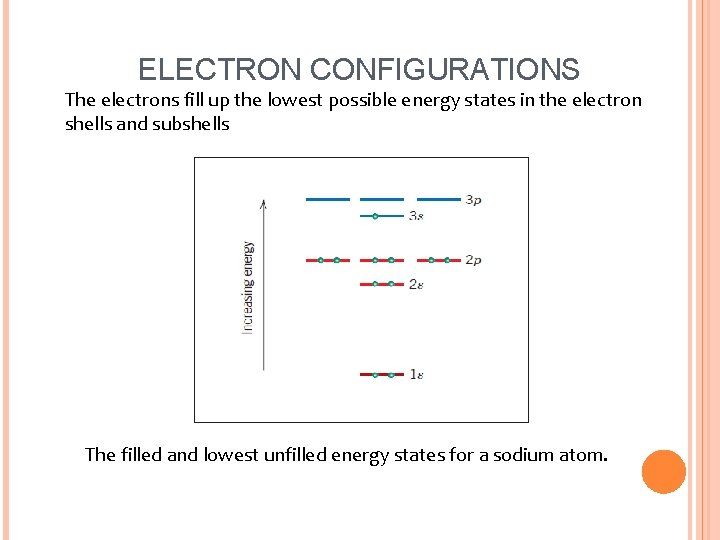
ELECTRON CONFIGURATIONS The electrons fill up the lowest possible energy states in the electron shells and subshells The filled and lowest unfilled energy states for a sodium atom.
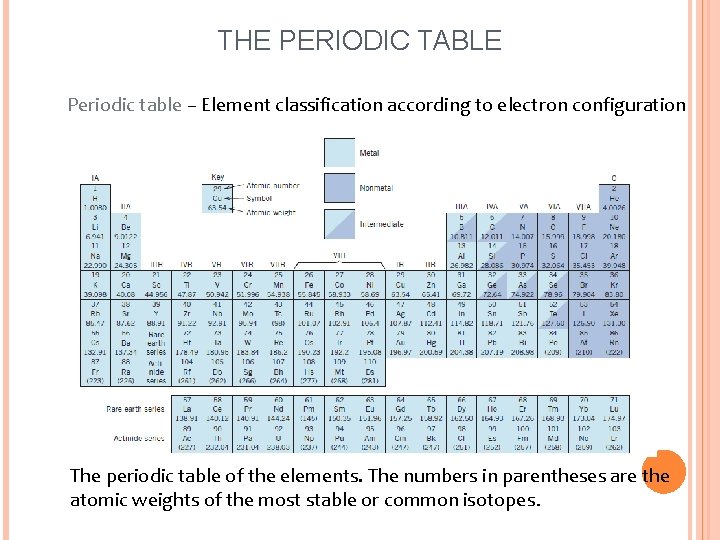
THE PERIODIC TABLE Periodic table – Element classification according to electron configuration The periodic table of the elements. The numbers in parentheses are the atomic weights of the most stable or common isotopes.
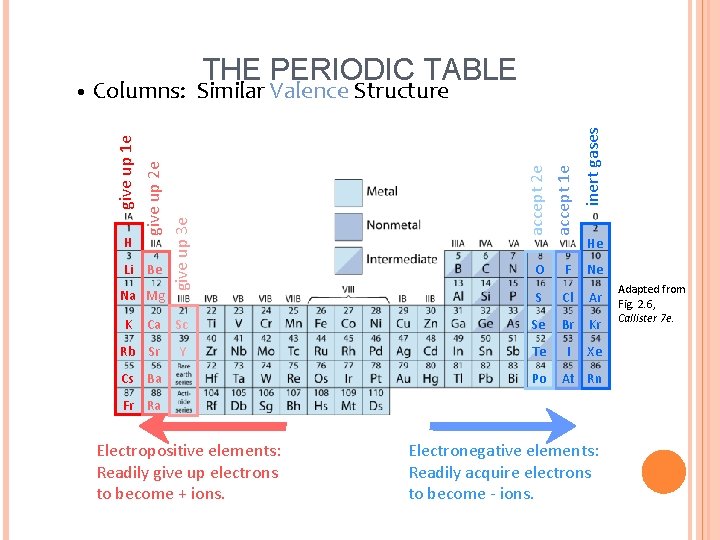
Li Be Na Mg K inert gases accept 2 e accept 1 e give up 3 e H give up 2 e Columns: Similar Valence Structure give up 1 e • THE PERIODIC TABLE He O F Ne S Cl Ar Ca Sc Se Br Kr Rb Sr Y Te I Xe Cs Ba Po At Rn Fr Ra Electropositive elements: Readily give up electrons to become + ions. Electronegative elements: Readily acquire electrons to become - ions. Adapted from Fig. 2. 6, Callister 7 e.
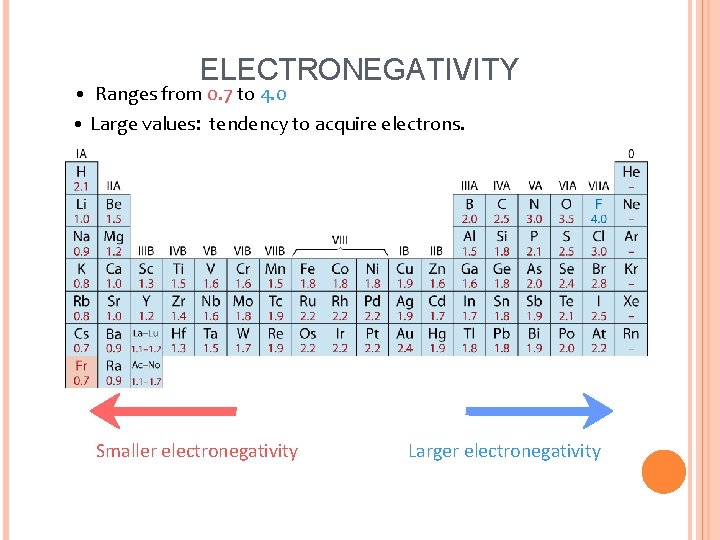
ELECTRONEGATIVITY • Ranges from 0. 7 to 4. 0 • Large values: tendency to acquire electrons. Smaller electronegativity Larger electronegativity
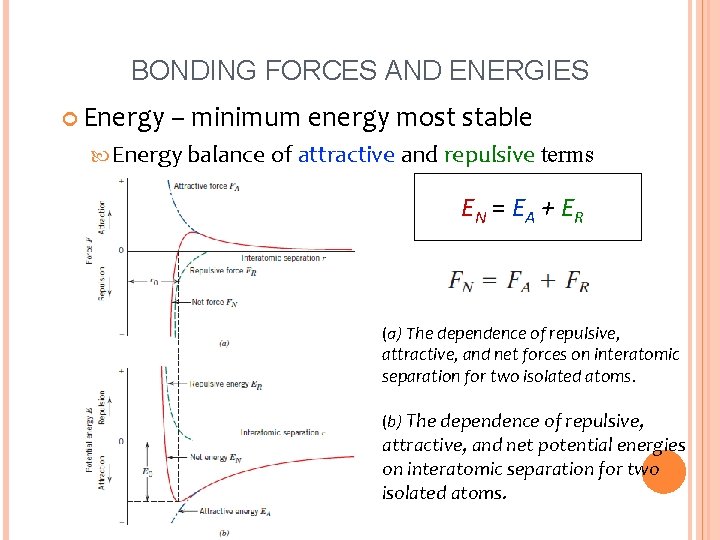
BONDING FORCES AND ENERGIES Energy – minimum energy most stable Energy balance of attractive and repulsive terms EN = EA + ER (a) The dependence of repulsive, attractive, and net forces on interatomic separation for two isolated atoms. (b) The dependence of repulsive, attractive, and net potential energies on interatomic separation for two isolated atoms.
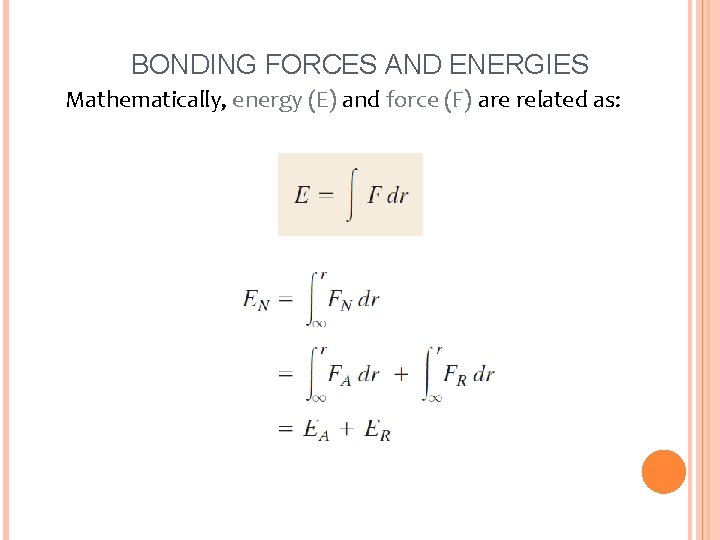
BONDING FORCES AND ENERGIES Mathematically, energy (E) and force (F) are related as:
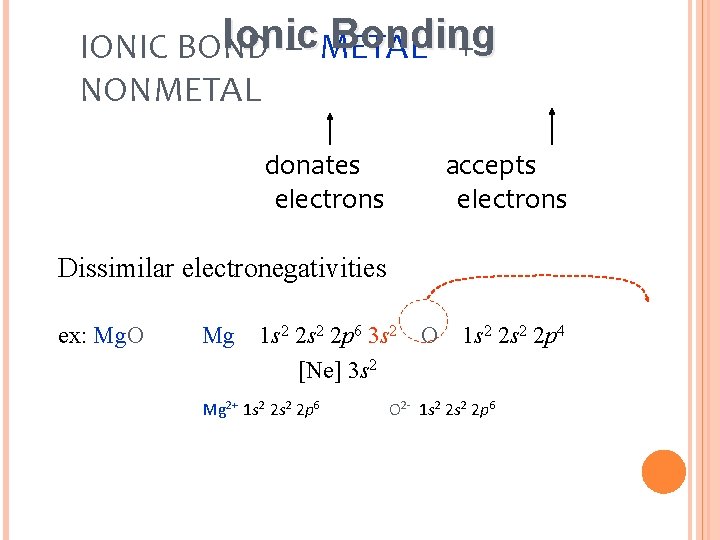
Ionic Bonding IONIC BOND – METAL + NONMETAL donates electrons accepts electrons Dissimilar electronegativities ex: Mg. O Mg 1 s 2 2 p 6 3 s 2 [Ne] 3 s 2 Mg 2+ 1 s 2 2 p 6 O 1 s 2 2 p 4 O 2 - 1 s 2 2 p 6
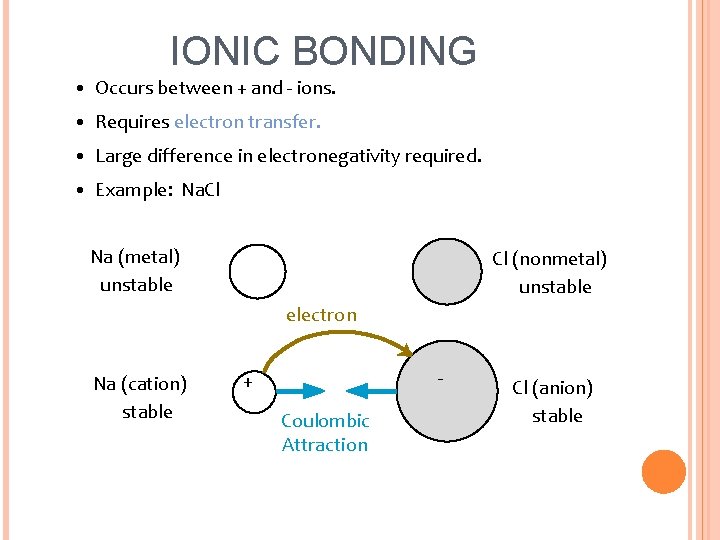
IONIC BONDING • Occurs between + and - ions. • Requires electron transfer. • Large difference in electronegativity required. • Example: Na. Cl Na (metal) unstable Cl (nonmetal) unstable electron Na (cation) stable - + Coulombic Attraction Cl (anion) stable
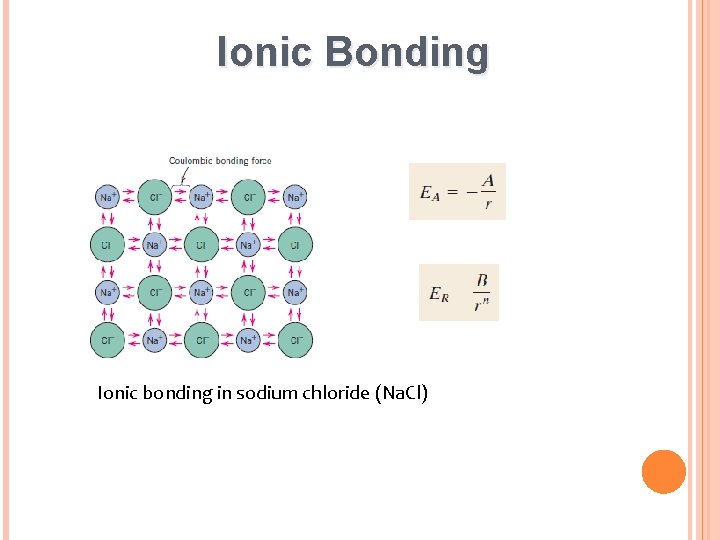
Ionic Bonding Ionic bonding in sodium chloride (Na. Cl)
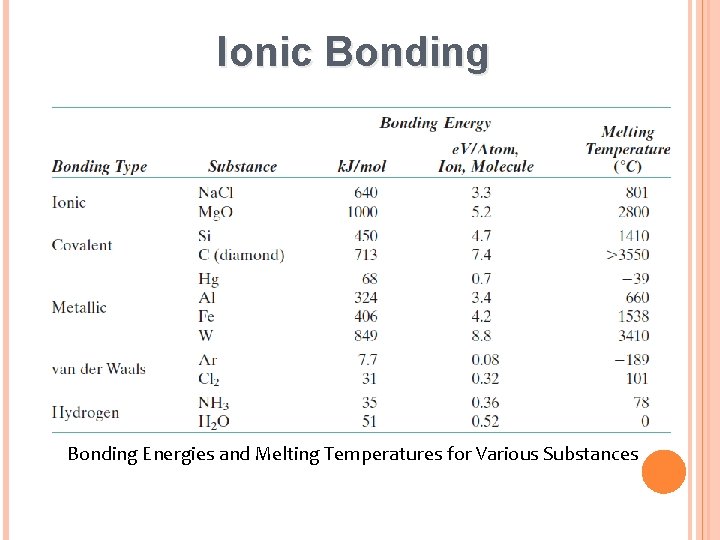
Ionic Bonding Energies and Melting Temperatures for Various Substances
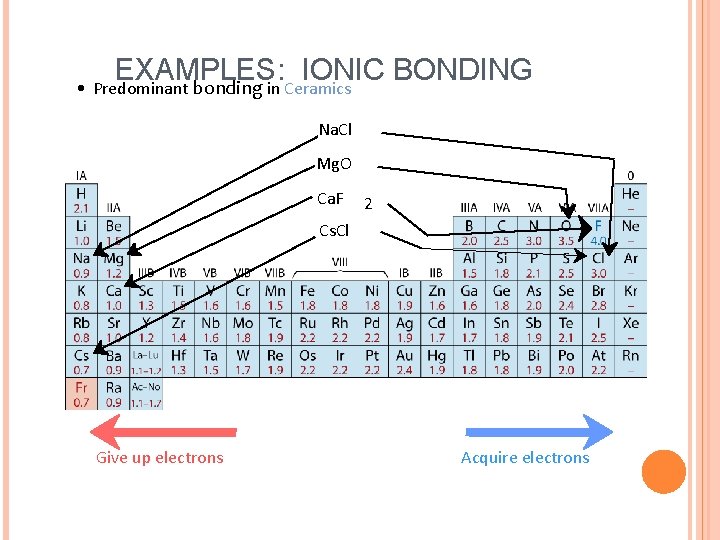
EXAMPLES: IONIC BONDING • Predominant bonding in Ceramics Na. Cl Mg. O Ca. F 2 Cs. Cl Give up electrons Acquire electrons
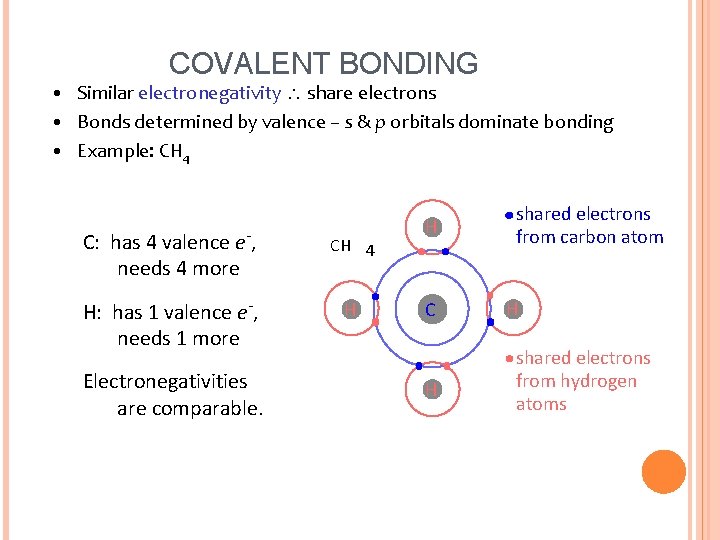
COVALENT BONDING • Similar electronegativity share electrons • Bonds determined by valence – s & p orbitals dominate bonding • Example: CH 4 C: has 4 valence e-, needs 4 more CH 4 H: has 1 valence e-, needs 1 more H Electronegativities are comparable. H C H shared electrons from carbon atom H shared electrons from hydrogen atoms
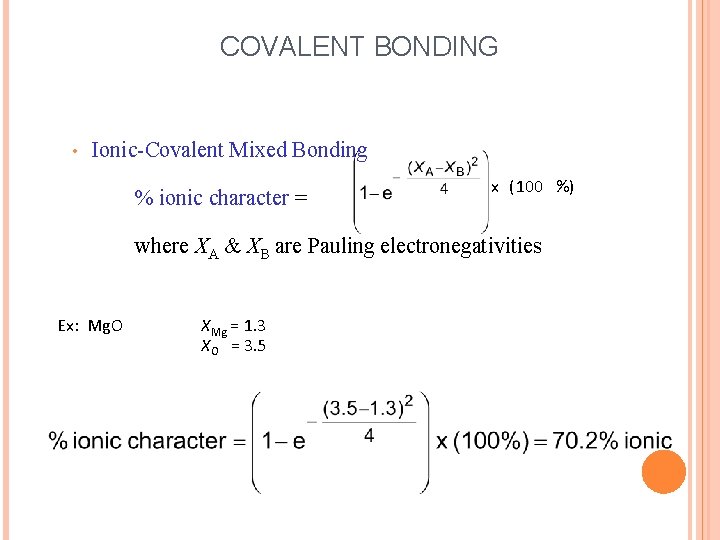
COVALENT BONDING • Ionic-Covalent Mixed Bonding % ionic character = x ( 100 %) where XA & XB are Pauling electronegativities Ex: Mg. O XMg = 1. 3 XO = 3. 5
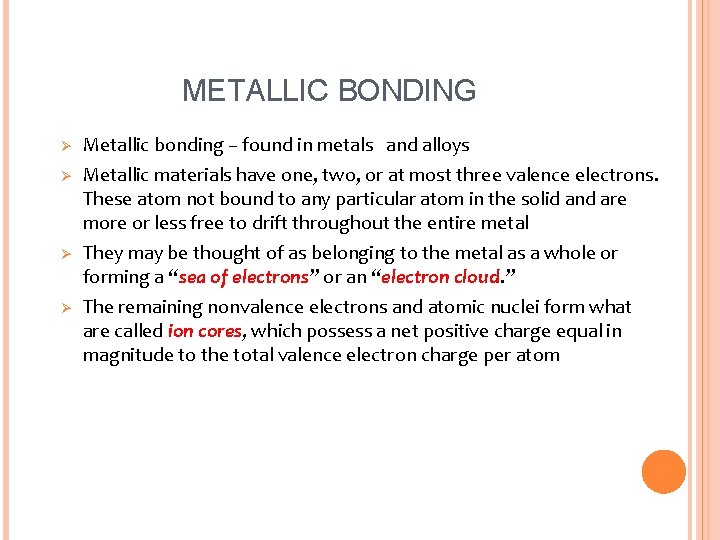
METALLIC BONDING Ø Metallic bonding – found in metals and alloys Ø Metallic materials have one, two, or at most three valence electrons. These atom not bound to any particular atom in the solid and are more or less free to drift throughout the entire metal They may be thought of as belonging to the metal as a whole or forming a “sea of electrons” or an “electron cloud. ” The remaining nonvalence electrons and atomic nuclei form what are called ion cores, which possess a net positive charge equal in magnitude to the total valence electron charge per atom Ø Ø
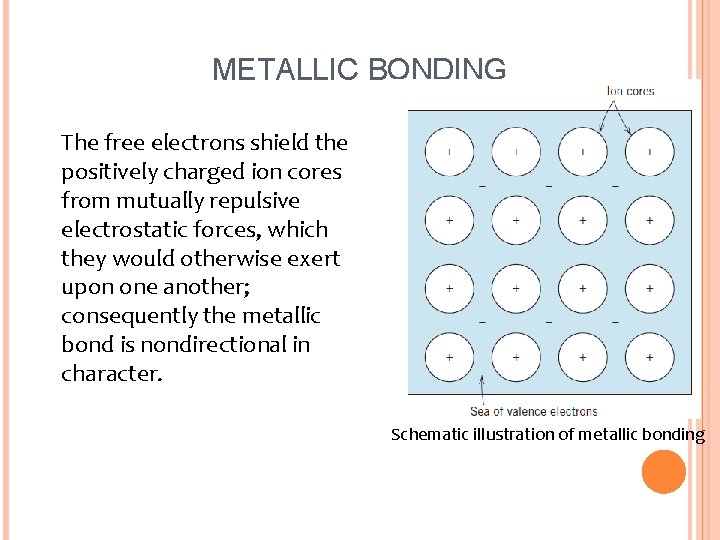
METALLIC BONDING The free electrons shield the positively charged ion cores from mutually repulsive electrostatic forces, which they would otherwise exert upon one another; consequently the metallic bond is nondirectional in character. Schematic illustration of metallic bonding
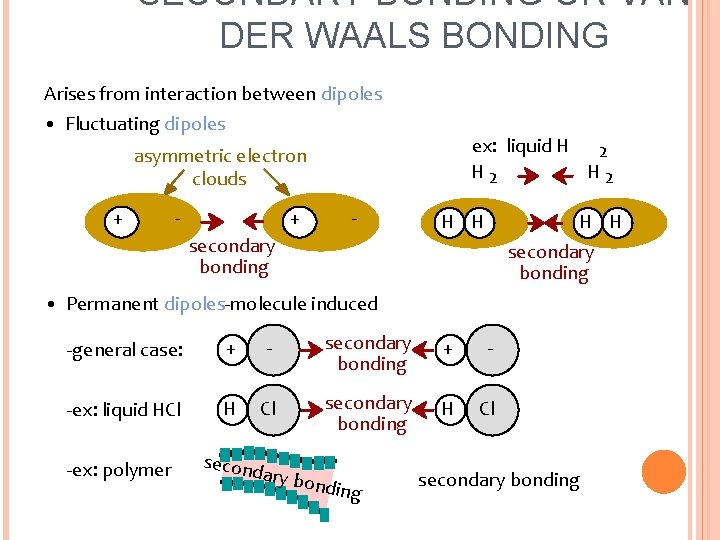
SECONDARY BONDING OR VAN DER WAALS BONDING Arises from interaction between dipoles • Fluctuating dipoles ex: liquid H 2 H 2 asymmetric electron clouds + - secondary bonding H H secondary bonding • Permanent dipoles-molecule induced -general case: + - secondary bonding + - -ex: liquid HCl H Cl secondary bonding H Cl -ex: polymer secon dary b ondin g secondary bonding
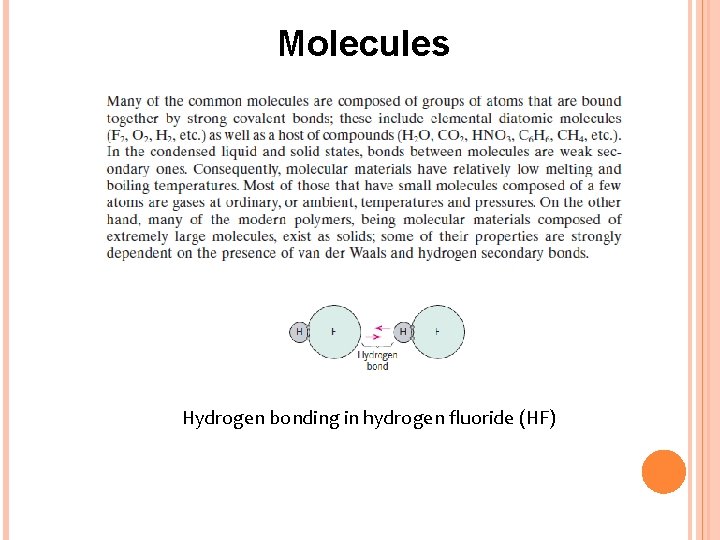
Molecules Hydrogen bonding in hydrogen fluoride (HF)
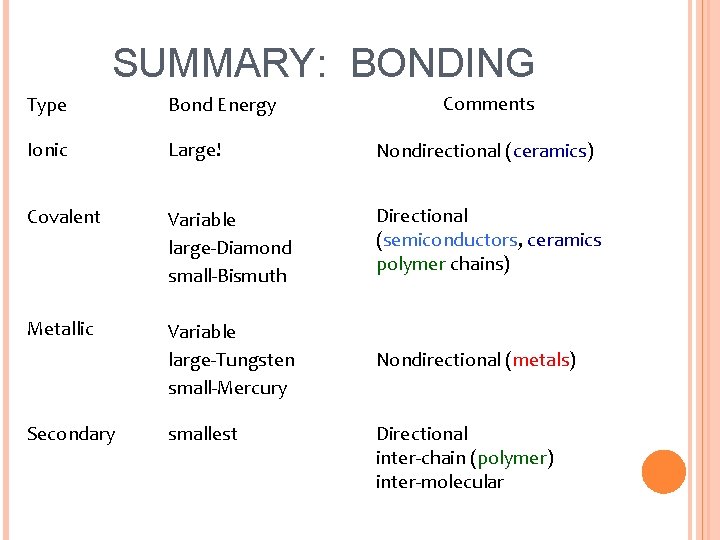
SUMMARY: BONDING Comments Type Bond Energy Ionic Large! Nondirectional (ceramics) Covalent Variable large-Diamond small-Bismuth Directional (semiconductors, ceramics polymer chains) Metallic Variable large-Tungsten small-Mercury Nondirectional (metals) Secondary smallest Directional inter-chain (polymer) inter-molecular
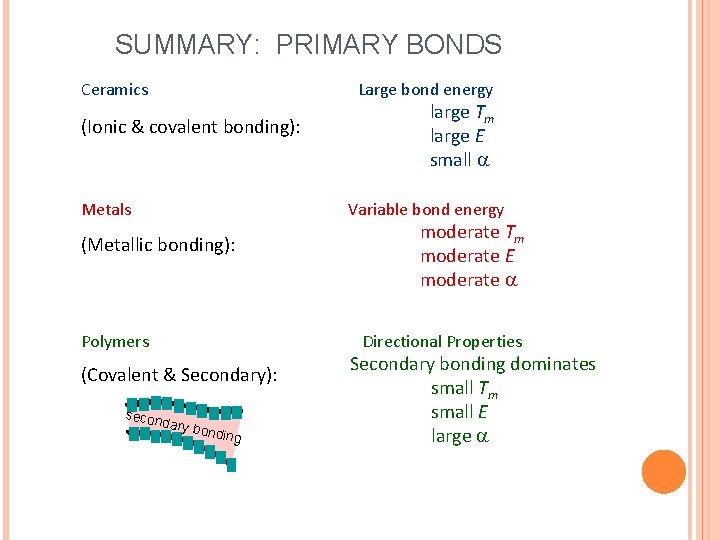
SUMMARY: PRIMARY BONDS Ceramics Large bond energy (Ionic & covalent bonding): Metals large Tm large E small Variable bond energy (Metallic bonding): Polymers moderate Tm moderate E moderate Directional Properties (Covalent & Secondary): secon dary b o nding Secondary bonding dominates small Tm small E large
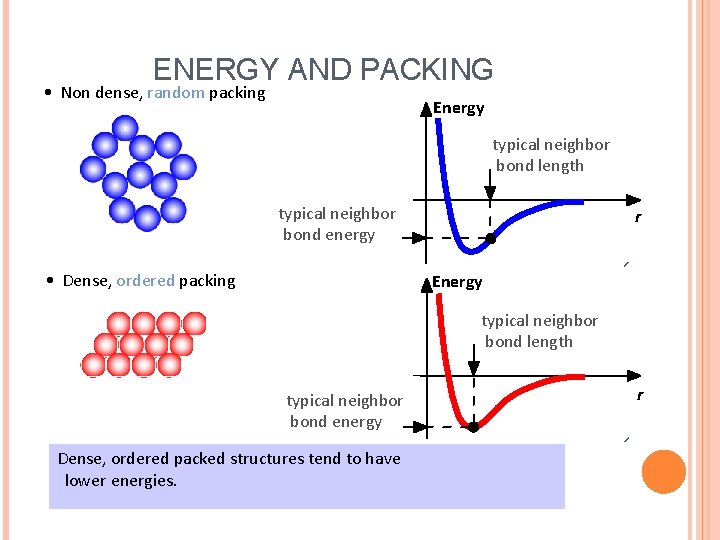
ENERGY AND PACKING • Non dense, random packing Energy typical neighbor bond length typical neighbor bond energy • Dense, ordered packing r Energy typical neighbor bond length typical neighbor bond energy Dense, ordered packed structures tend to have lower energies. r
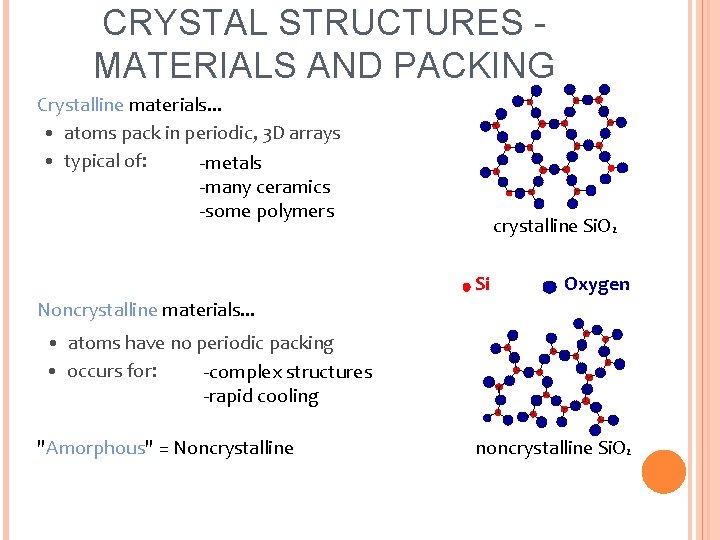
CRYSTAL STRUCTURES MATERIALS AND PACKING Crystalline materials. . . • atoms pack in periodic, 3 D arrays • typical of: -metals -many ceramics -some polymers crystalline Si. O 2 Si Oxygen Noncrystalline materials. . . • atoms have no periodic packing • occurs for: -complex structures -rapid cooling "Amorphous" = Noncrystalline noncrystalline Si. O 2
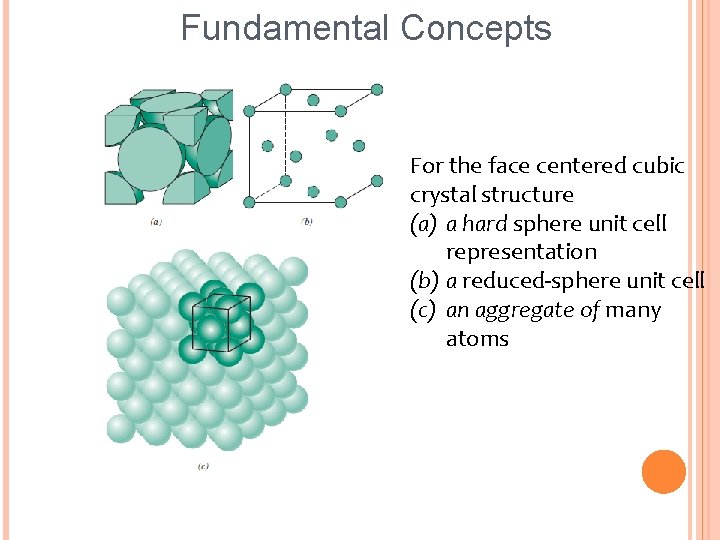
Fundamental Concepts For the face centered cubic crystal structure (a) a hard sphere unit cell representation (b) a reduced-sphere unit cell (c) an aggregate of many atoms
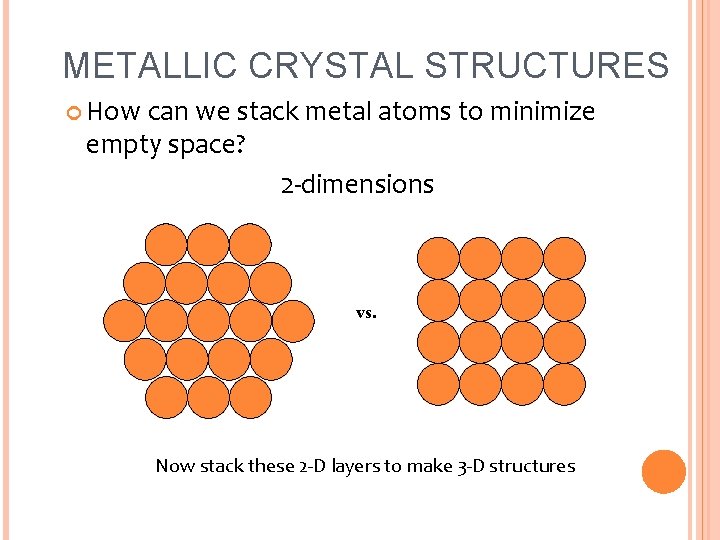
METALLIC CRYSTAL STRUCTURES How can we stack metal atoms to minimize empty space? 2 -dimensions vs. Now stack these 2 -D layers to make 3 -D structures
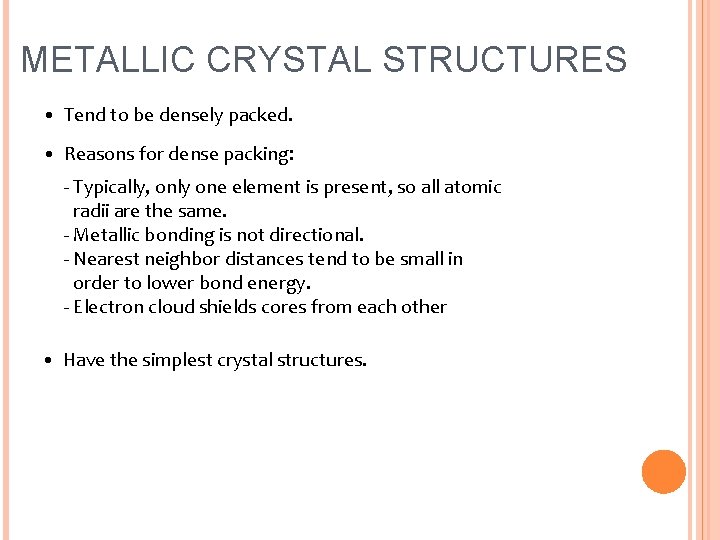
METALLIC CRYSTAL STRUCTURES • Tend to be densely packed. • Reasons for dense packing: - Typically, only one element is present, so all atomic radii are the same. - Metallic bonding is not directional. - Nearest neighbor distances tend to be small in order to lower bond energy. - Electron cloud shields cores from each other • Have the simplest crystal structures.
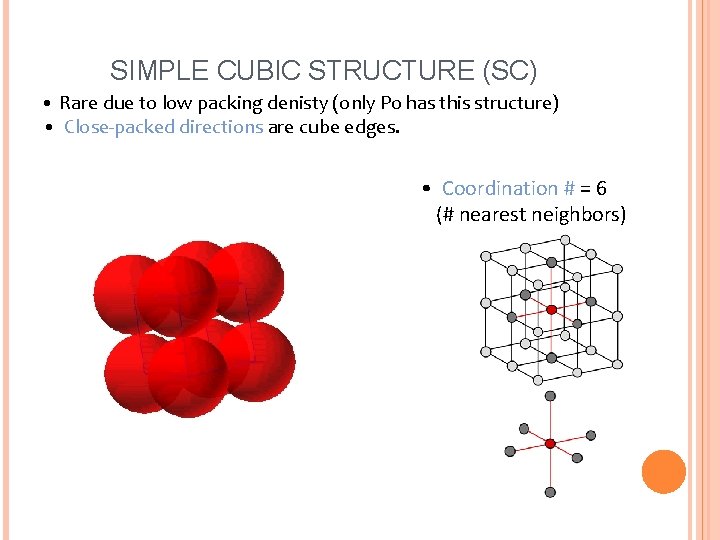
SIMPLE CUBIC STRUCTURE (SC) • Rare due to low packing denisty (only Po has this structure) • Close-packed directions are cube edges. • Coordination # = 6 (# nearest neighbors)
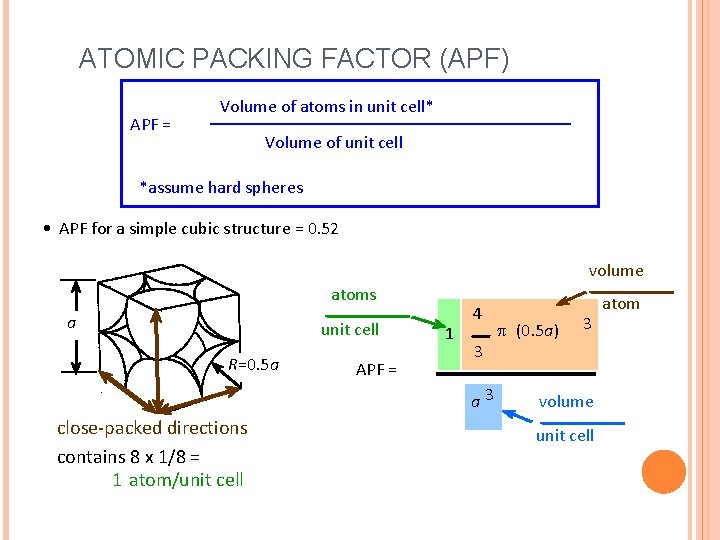
ATOMIC PACKING FACTOR (APF) APF = Volume of atoms in unit cell* Volume of unit cell *assume hard spheres • APF for a simple cubic structure = 0. 52 volume atoms a unit cell R=0. 5 a APF = 1 4 3 a 3 close-packed directions contains 8 x 1/8 = 1 atom/unit cell p (0. 5 a) 3 volume unit cell atom
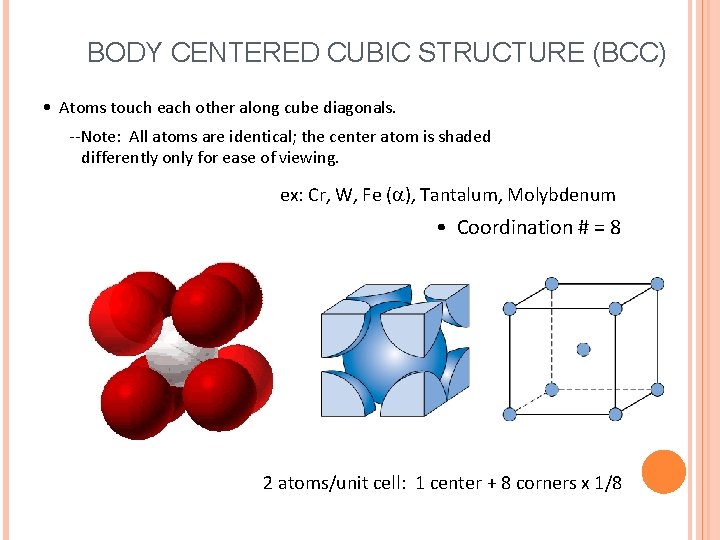
BODY CENTERED CUBIC STRUCTURE (BCC) • Atoms touch each other along cube diagonals. --Note: All atoms are identical; the center atom is shaded differently only for ease of viewing. ex: Cr, W, Fe ( ), Tantalum, Molybdenum • Coordination # = 8 2 atoms/unit cell: 1 center + 8 corners x 1/8
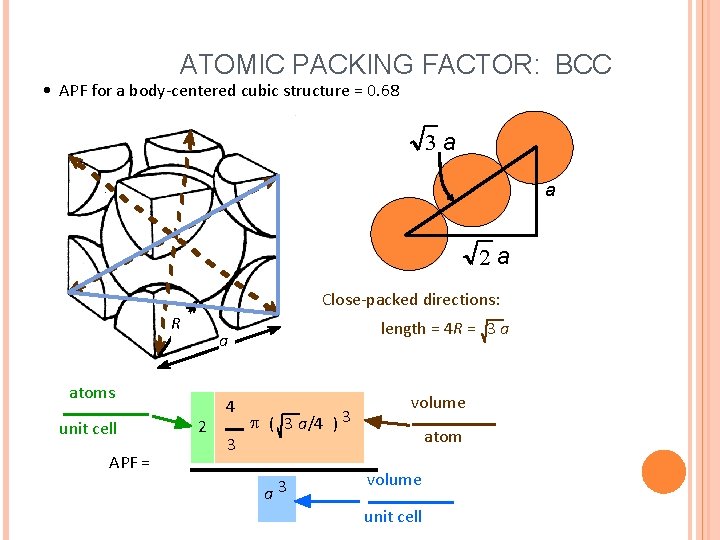
ATOMIC PACKING FACTOR: BCC • APF for a body-centered cubic structure = 0. 68 3 a a 2 a Close-packed directions: R atoms unit cell APF = length = 4 R = 3 a a 2 4 3 p ( 3 a/4 ) 3 a 3 volume atom volume unit cell
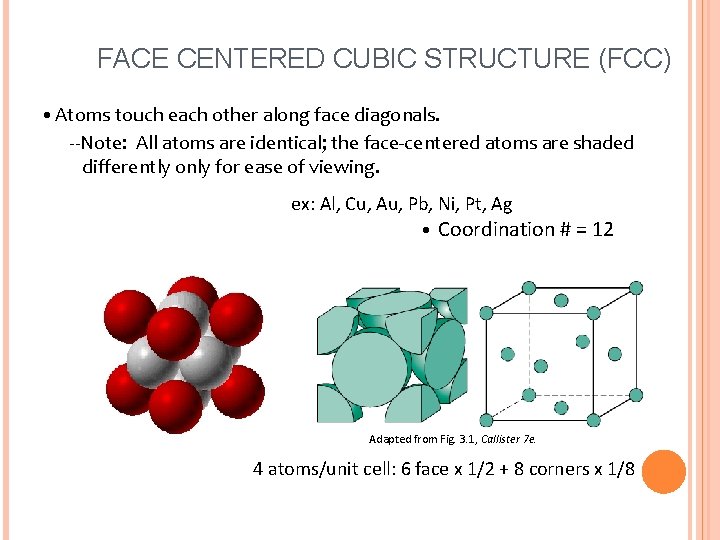
FACE CENTERED CUBIC STRUCTURE (FCC) • Atoms touch each other along face diagonals. --Note: All atoms are identical; the face-centered atoms are shaded differently only for ease of viewing. ex: Al, Cu, Au, Pb, Ni, Pt, Ag • Coordination # = 12 Adapted from Fig. 3. 1, Callister 7 e. 4 atoms/unit cell: 6 face x 1/2 + 8 corners x 1/8
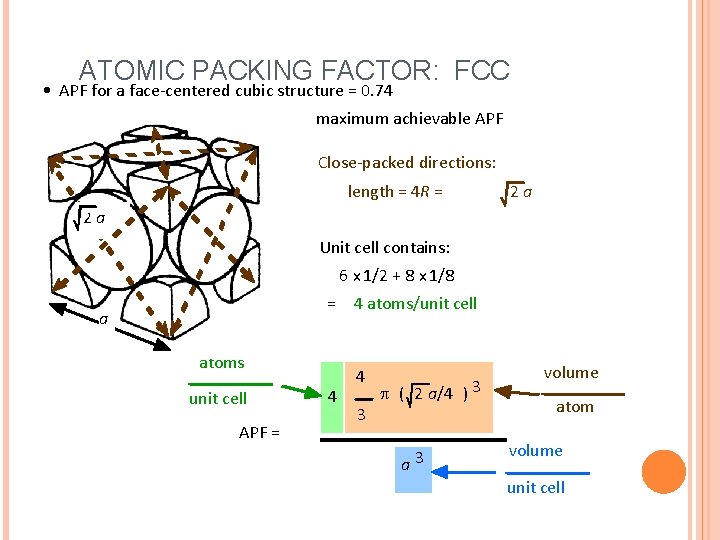
ATOMIC PACKING FACTOR: FCC • APF for a face-centered cubic structure = 0. 74 maximum achievable APF Close-packed directions: length = 4 R = 2 a 2 a Unit cell contains: 6 x 1/2 + 8 x 1/8 = 4 atoms/unit cell a atoms unit cell APF = 4 4 3 p ( 2 a/4 ) 3 a 3 volume atom volume unit cell
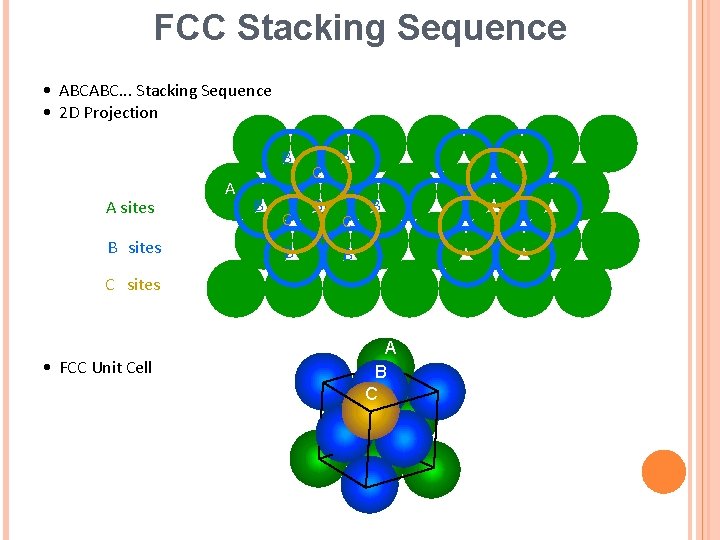
FCC Stacking Sequence • ABCABC. . . Stacking Sequence • 2 D Projection B A sites B sites A B C B B C sites • FCC Unit Cell A B C
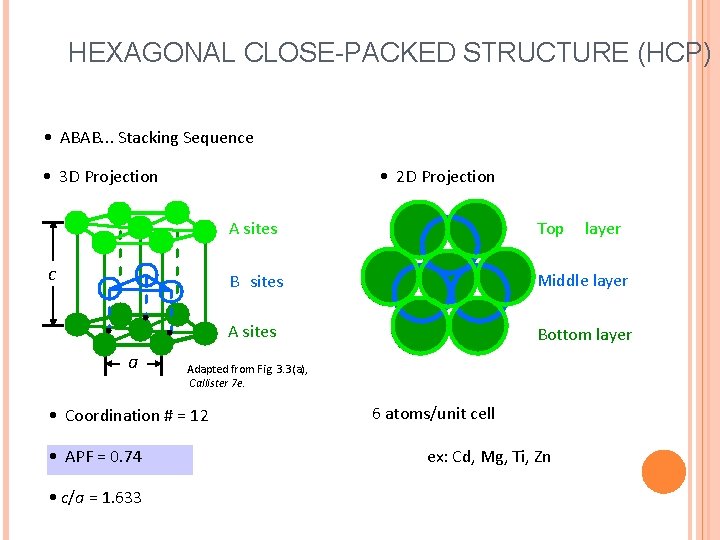
HEXAGONAL CLOSE-PACKED STRUCTURE (HCP) • ABAB. . . Stacking Sequence • 3 D Projection • 2 D Projection c a • c/a = 1. 633 Top B sites Middle layer A sites Bottom layer Adapted from Fig. 3. 3(a), Callister 7 e. • Coordination # = 12 • APF = 0. 74 A sites 6 atoms/unit cell ex: Cd, Mg, Ti, Zn layer
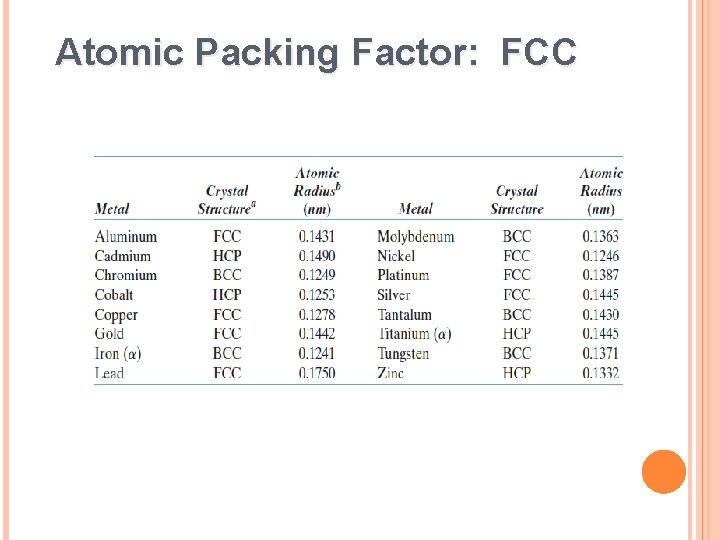
Atomic Packing Factor: FCC
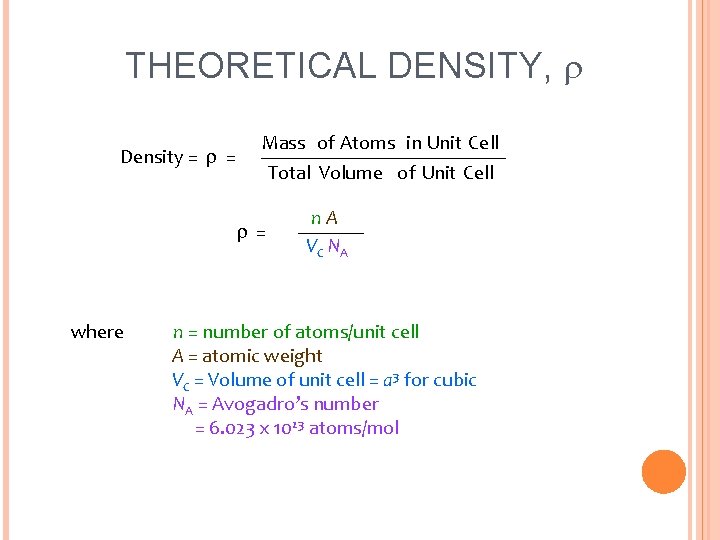
THEORETICAL DENSITY, Density = = Mass of Atoms in Unit Cell Total Volume of Unit Cell = where n. A VC NA n = number of atoms/unit cell A = atomic weight VC = Volume of unit cell = a 3 for cubic NA = Avogadro’s number = 6. 023 x 1023 atoms/mol
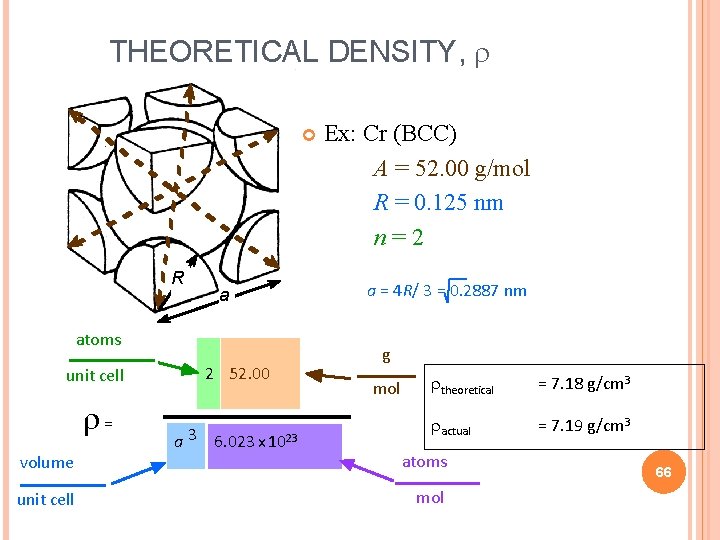
THEORETICAL DENSITY, R a atoms unit cell = volume unit cell 2 52. 00 a 3 6. 023 x 1023 Ex: Cr (BCC) A = 52. 00 g/mol R = 0. 125 nm n=2 a = 4 R/ 3 = 0. 2887 nm g mol theoretical = 7. 18 g/cm 3 actual = 7. 19 g/cm 3 atoms mol 66
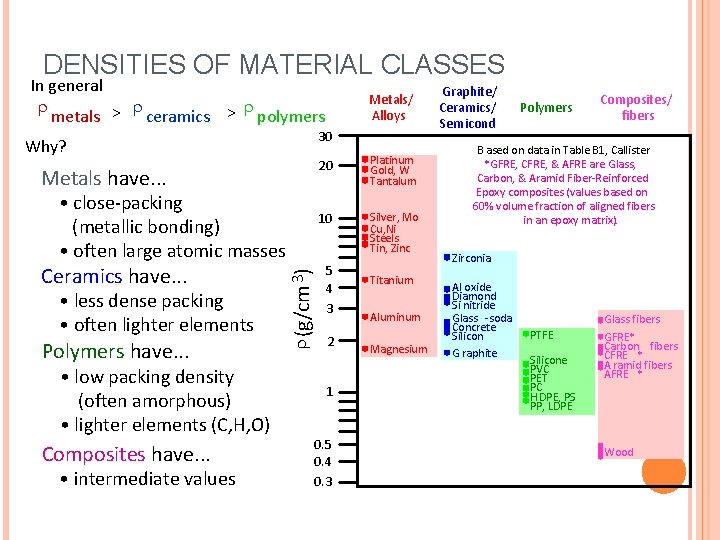
DENSITIES OF MATERIAL CLASSES In general metals > ceramics > polymers 30 Why? Metals have. . . • less dense packing • often lighter elements Polymers have. . . • low packing density (often amorphous) • lighter elements (C, H, O) Composites have. . . • intermediate values (g/cm 3) • close-packing (metallic bonding) • often large atomic masses Ceramics have. . . Metals/ Alloys 20 Platinum Gold, W Tantalum 10 Silver, Mo Cu, Ni Steels Tin, Zinc 5 4 3 2 1 0. 5 0. 4 0. 3 Titanium Aluminum Magnesium Graphite/ Ceramics/ Semicond Polymers Composites/ fibers B ased on data in Table B 1, Callister *GFRE, CFRE, & AFRE are Glass, Carbon, & Aramid Fiber-Reinforced Epoxy composites (values based on 60% volume fraction of aligned fibers in an epoxy matrix). Zirconia Al oxide Diamond Si nitride Glass -soda Concrete Silicon G raphite PTFE Silicone PVC PET PC H DPE, PS PP, LDPE Glass fibers GFRE* Carbon fibers CFRE * A ramid fibers AFRE * Wood
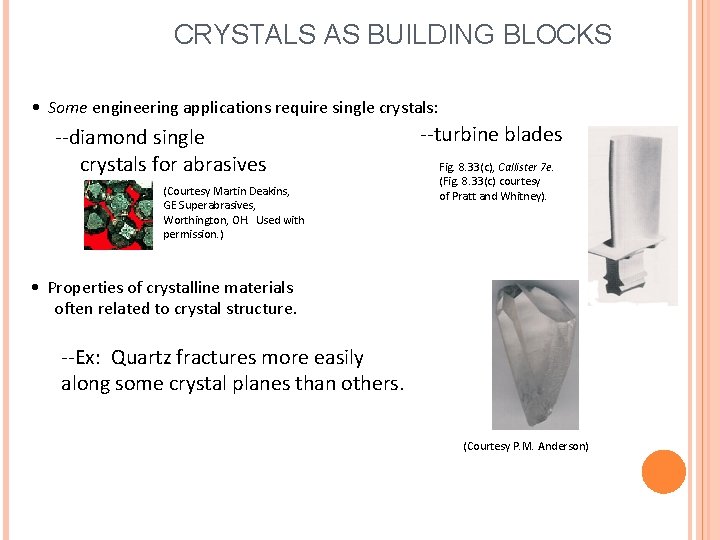
CRYSTALS AS BUILDING BLOCKS • Some engineering applications require single crystals: --diamond single crystals for abrasives (Courtesy Martin Deakins, GE Superabrasives, Worthington, OH. Used with permission. ) --turbine blades Fig. 8. 33(c), Callister 7 e. (Fig. 8. 33(c) courtesy of Pratt and Whitney). • Properties of crystalline materials often related to crystal structure. --Ex: Quartz fractures more easily along some crystal planes than others. (Courtesy P. M. Anderson)
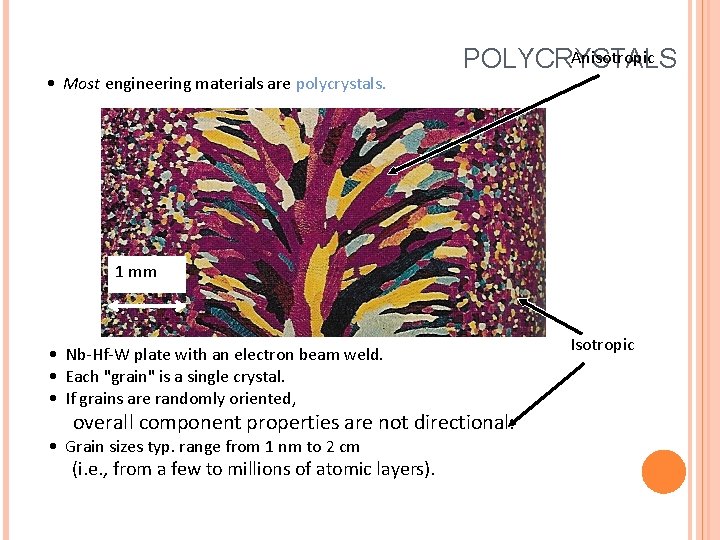
• Most engineering materials are polycrystals. Anisotropic POLYCRYSTALS 1 mm • Nb-Hf-W plate with an electron beam weld. • Each "grain" is a single crystal. • If grains are randomly oriented, overall component properties are not directional. • Grain sizes typ. range from 1 nm to 2 cm (i. e. , from a few to millions of atomic layers). Isotropic
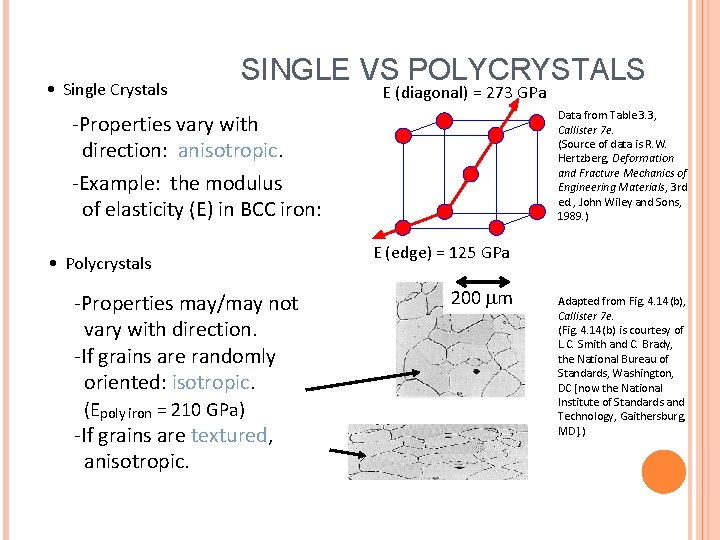
• Single Crystals SINGLE VS POLYCRYSTALS E (diagonal) = 273 GPa Data from Table 3. 3, Callister 7 e. (Source of data is R. W. Hertzberg, Deformation and Fracture Mechanics of Engineering Materials, 3 rd ed. , John Wiley and Sons, 1989. ) -Properties vary with direction: anisotropic. -Example: the modulus of elasticity (E) in BCC iron: • Polycrystals -Properties may/may not vary with direction. -If grains are randomly oriented: isotropic. (Epoly iron = 210 GPa) -If grains are textured, anisotropic. E (edge) = 125 GPa 200 mm Adapted from Fig. 4. 14(b), Callister 7 e. (Fig. 4. 14(b) is courtesy of L. C. Smith and C. Brady, the National Bureau of Standards, Washington, DC [now the National Institute of Standards and Technology, Gaithersburg, MD]. )
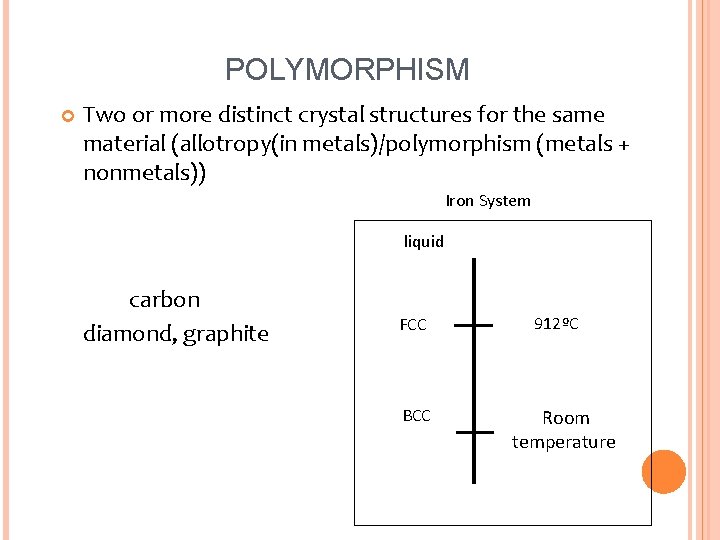
POLYMORPHISM Two or more distinct crystal structures for the same material (allotropy(in metals)/polymorphism (metals + nonmetals)) Iron System liquid carbon diamond, graphite FCC BCC 912ºC Room temperature
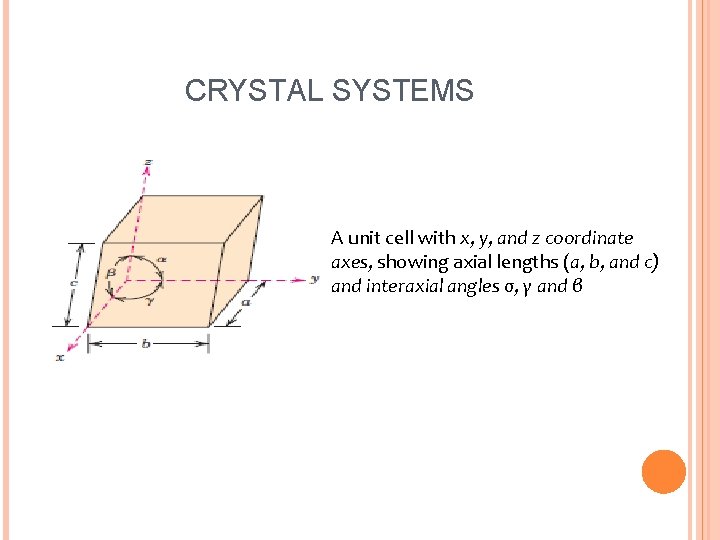
CRYSTAL SYSTEMS A unit cell with x, y, and z coordinate axes, showing axial lengths (a, b, and c) and interaxial angles σ, γ and β
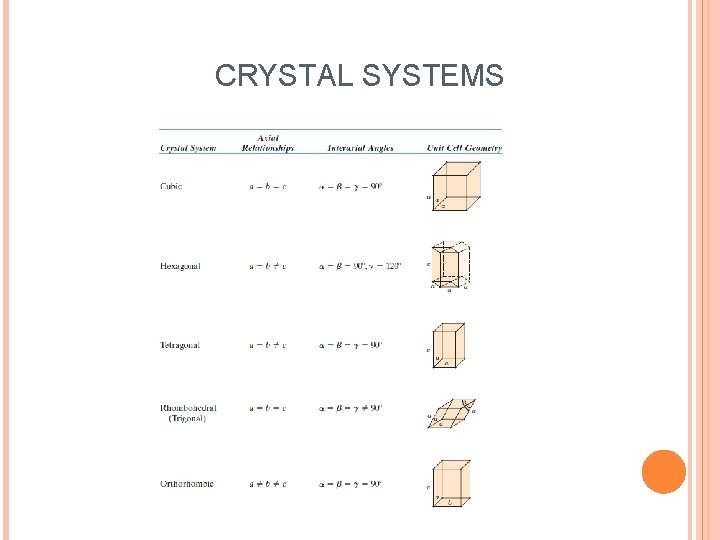
CRYSTAL SYSTEMS
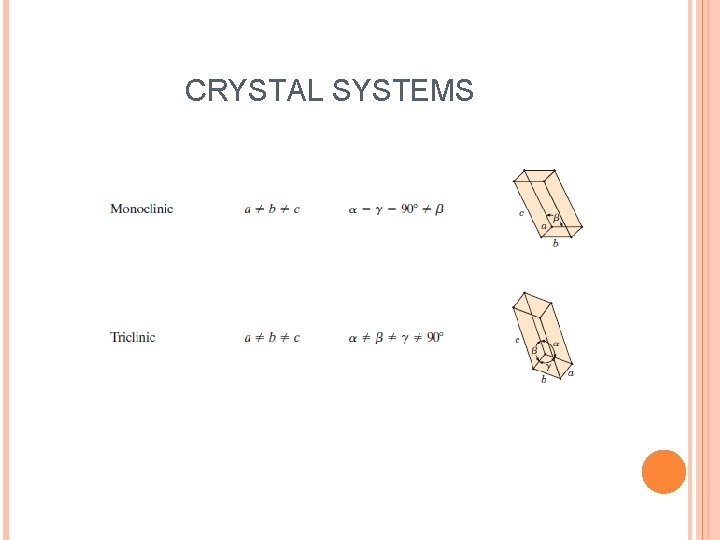
CRYSTAL SYSTEMS
Football heart
Dr. martin rovers
Courcey rovers
Come holy spirit, come hymn
Spirit spirit of gentleness
Gd and t symbols
Standard costing and variance analysis formula
Time space compression ap human geography
Examples of non material culture
Examples of non material culture
Useful and harmful
Iit delhi material science
Hình ảnh bộ gõ cơ thể búng tay
Lp html
Bổ thể
Tỉ lệ cơ thể trẻ em
Gấu đi như thế nào
Tư thế worm breton
Chúa yêu trần thế alleluia
Môn thể thao bắt đầu bằng chữ đua
Thế nào là hệ số cao nhất
Các châu lục và đại dương trên thế giới
Công thức tiính động năng
Trời xanh đây là của chúng ta thể thơ
Mật thư tọa độ 5x5
101012 bằng
Phản ứng thế ankan
Các châu lục và đại dương trên thế giới
Thơ thất ngôn tứ tuyệt đường luật
Quá trình desamine hóa có thể tạo ra
Một số thể thơ truyền thống
Cái miệng nó xinh thế chỉ nói điều hay thôi
Vẽ hình chiếu vuông góc của vật thể sau
Thế nào là sự mỏi cơ
đặc điểm cơ thể của người tối cổ
V cc cc
Vẽ hình chiếu đứng bằng cạnh của vật thể
Phối cảnh
Thẻ vin
đại từ thay thế
điện thế nghỉ
Tư thế ngồi viết
Diễn thế sinh thái là
Dạng đột biến một nhiễm là
Số nguyên tố là số gì
Tư thế ngồi viết
Lời thề hippocrates
Thiếu nhi thế giới liên hoan
ưu thế lai là gì
Khi nào hổ mẹ dạy hổ con săn mồi
Sự nuôi và dạy con của hươu
Sơ đồ cơ thể người
Từ ngữ thể hiện lòng nhân hậu
Thế nào là mạng điện lắp đặt kiểu nổi
Material handling introduction
Product handling definition
System architecture example
Forward engineering in software engineering
Dicapine
Elegant systems
Reverse engineering vs forward engineering
Transmission ciblée exemple
Mars society logo
Stoa speech ranks
Wenus i mars botticelli
Mars retrograde motion animation
Mars april mai
Sermon on mars hill
Mars radius
Physical characteristics of mars
Mars gerakan pramuka
Mengenangkan nasib perjuangan
Mars god symbols
Mars exploration program analysis group
Fun facts about mars