Energy and the New Reality Volume 1 Energy
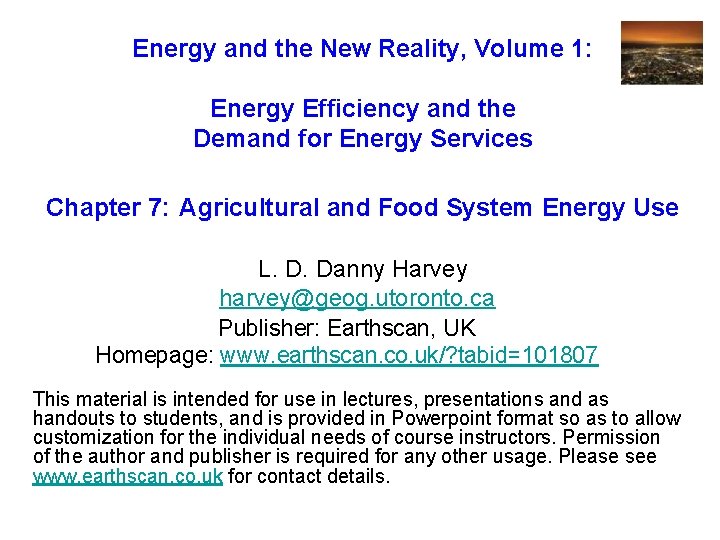
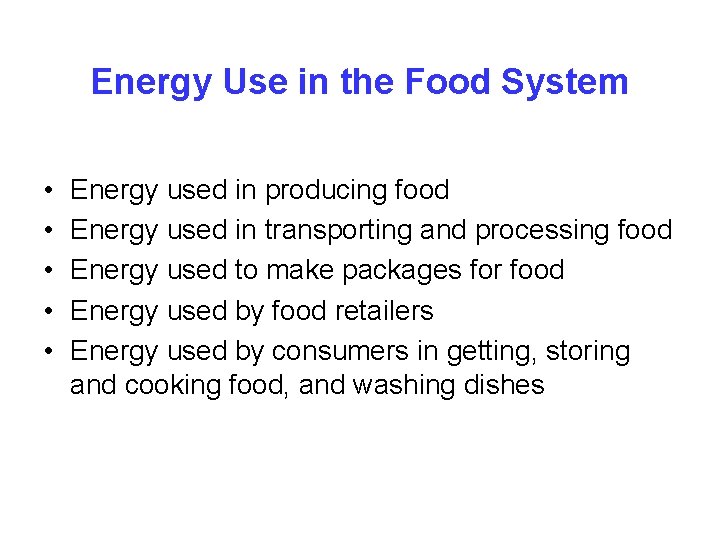
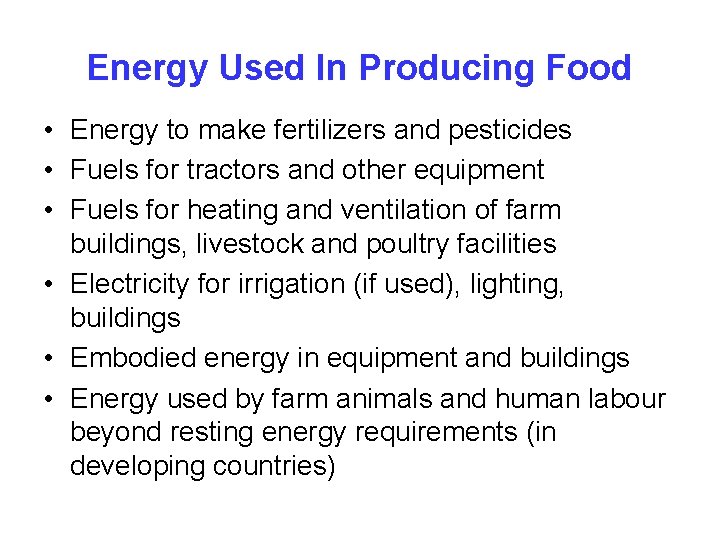
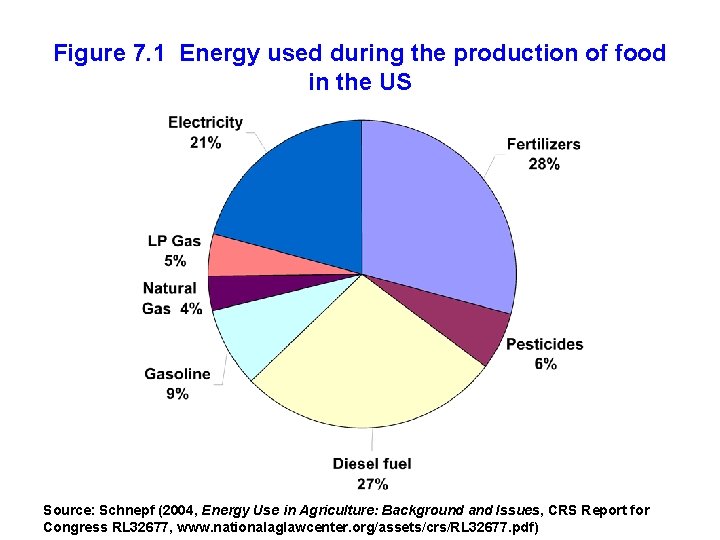
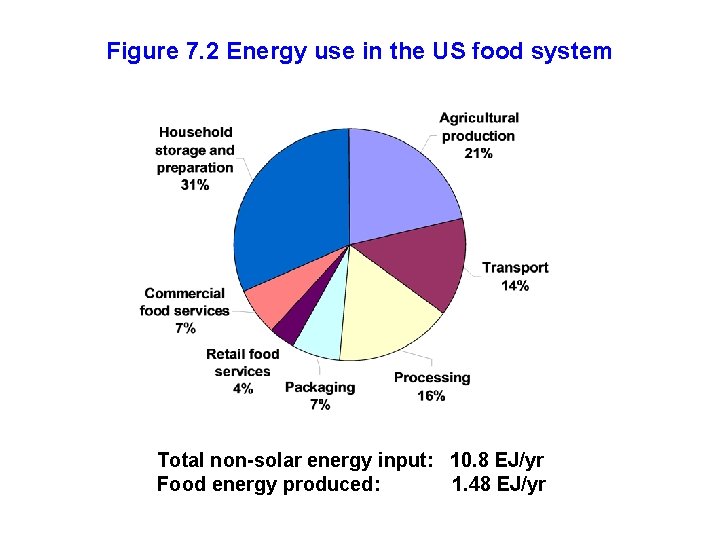
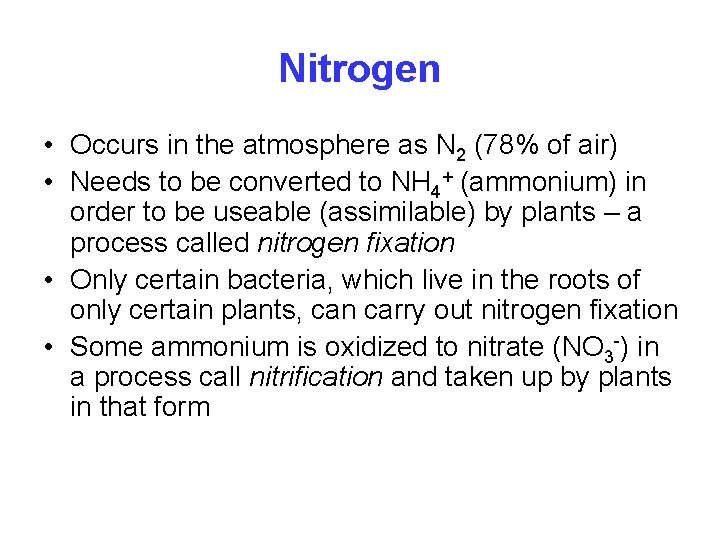
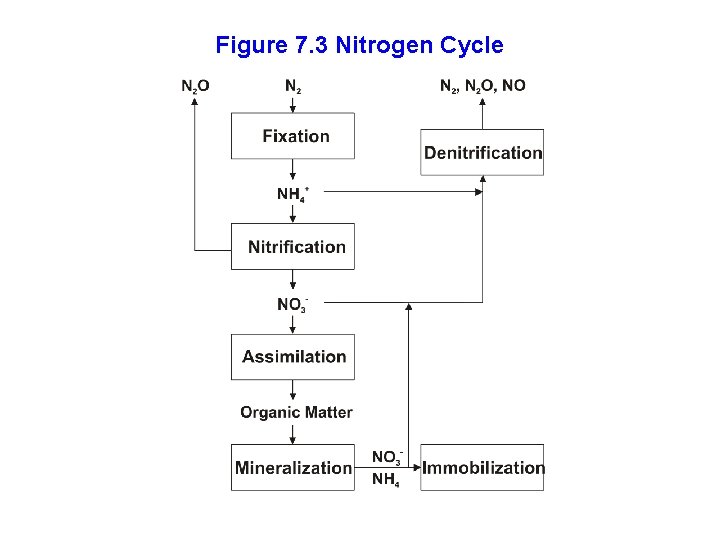
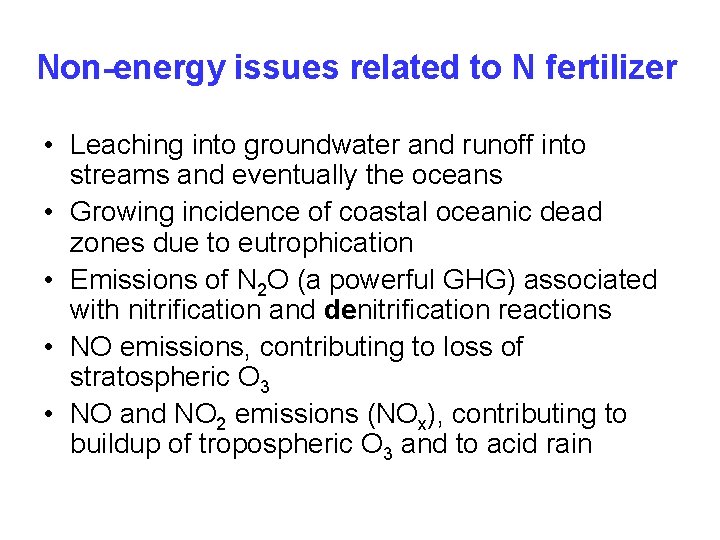
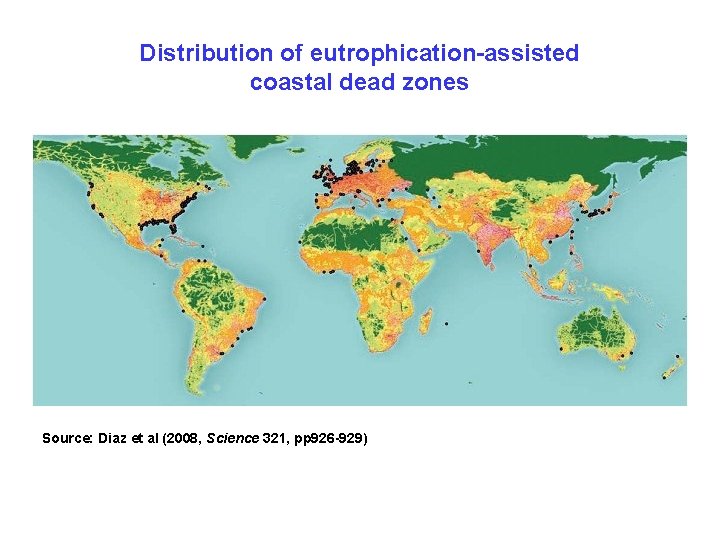
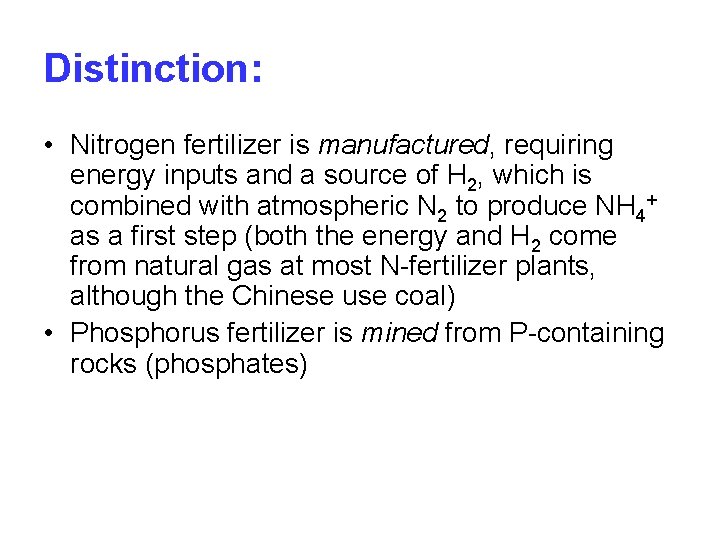
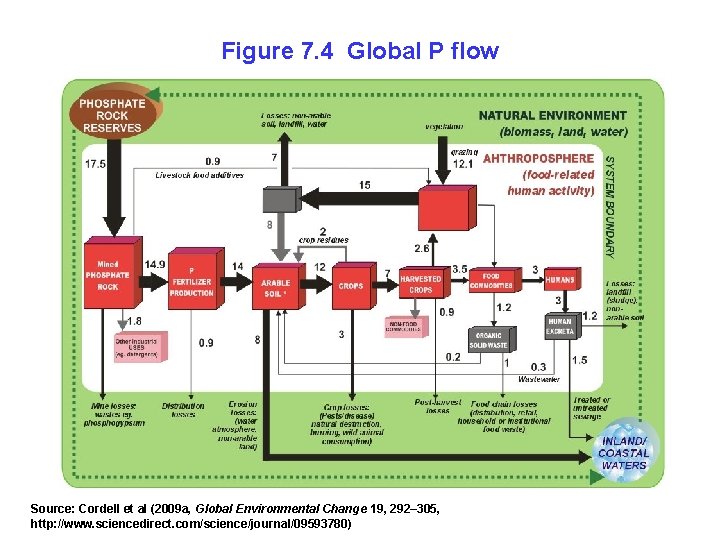
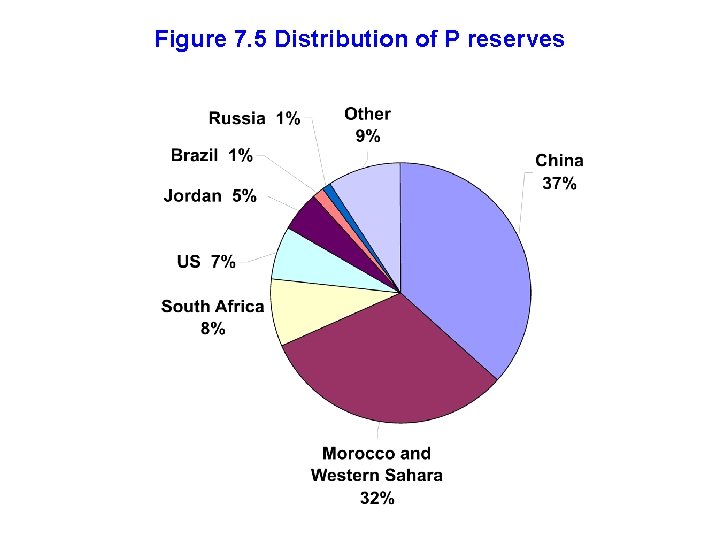
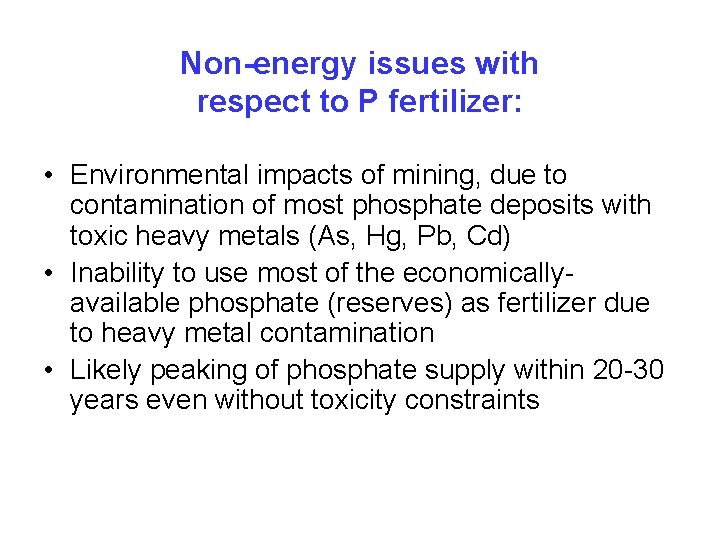
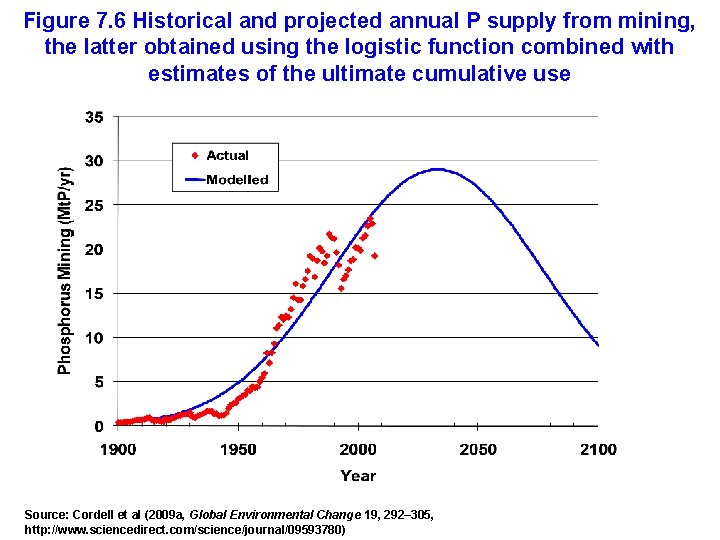
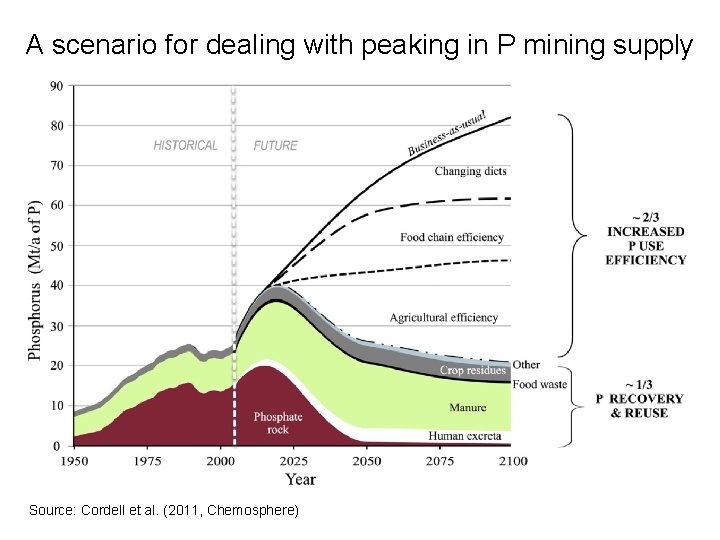
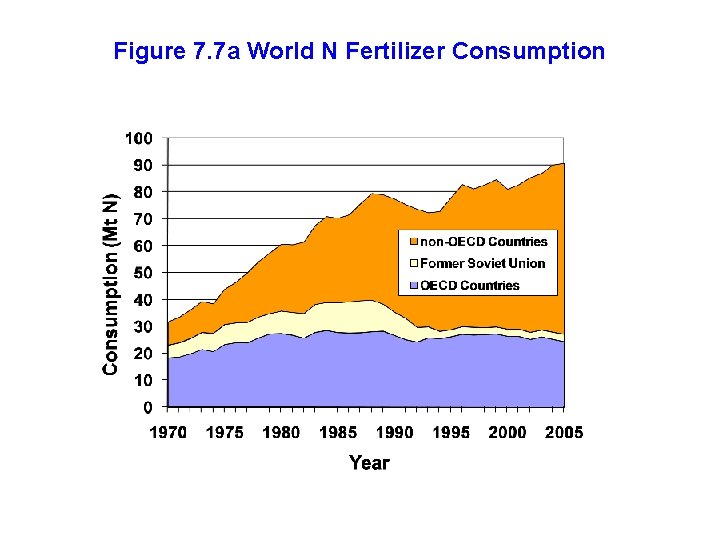
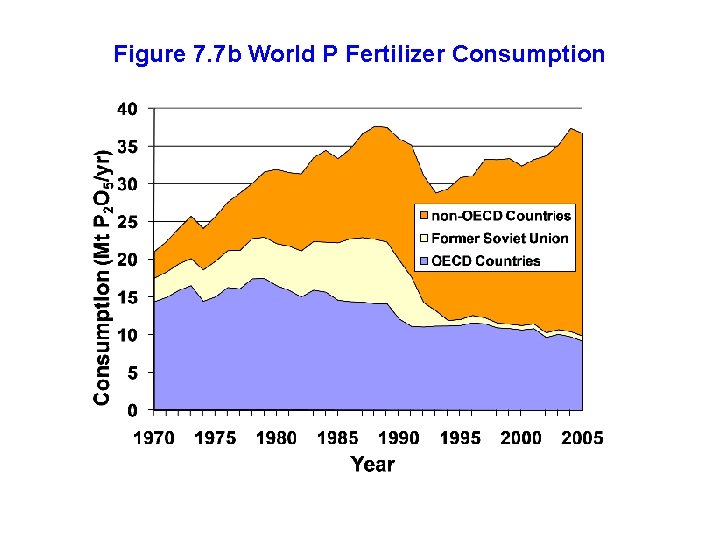
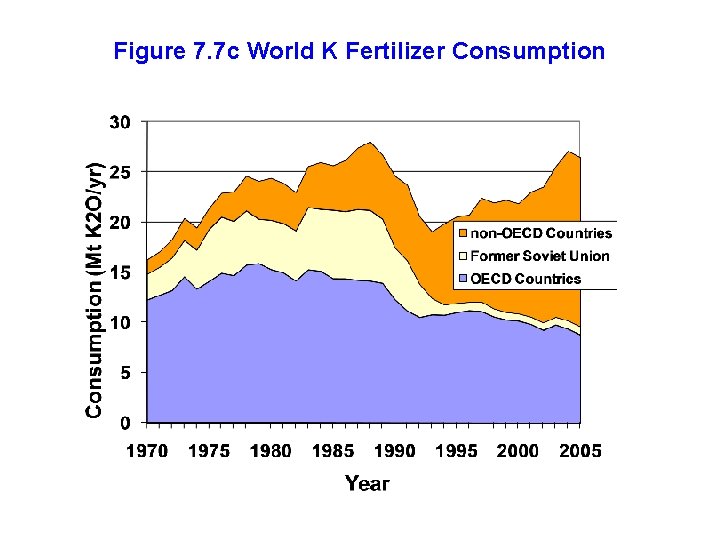
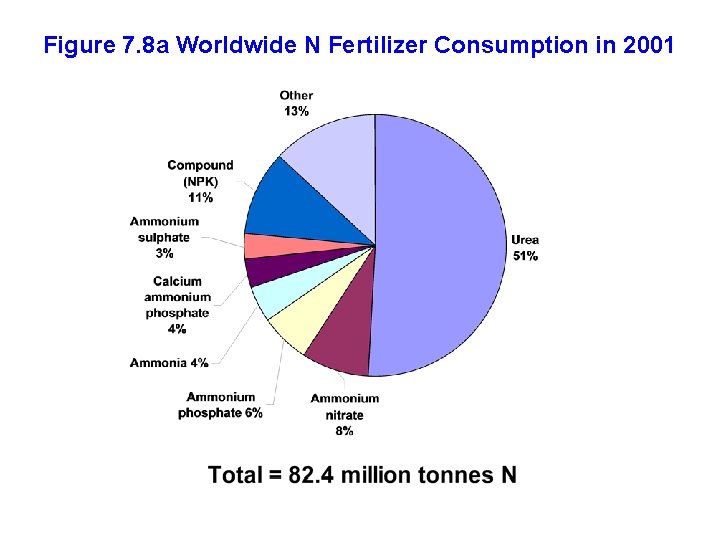
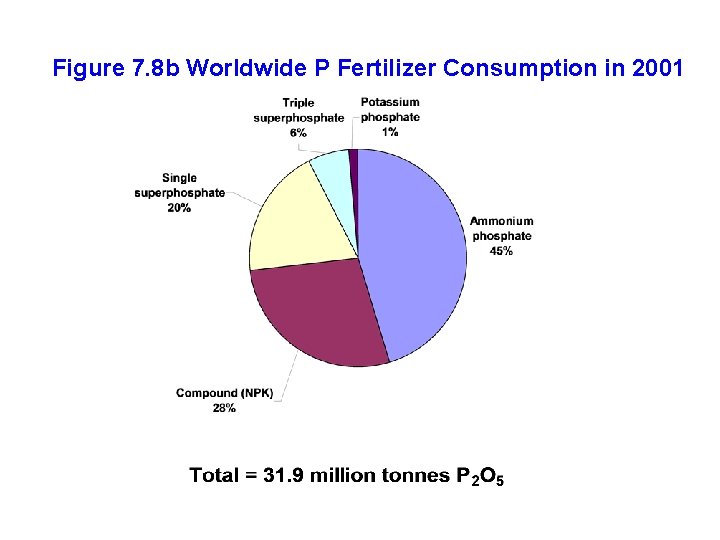
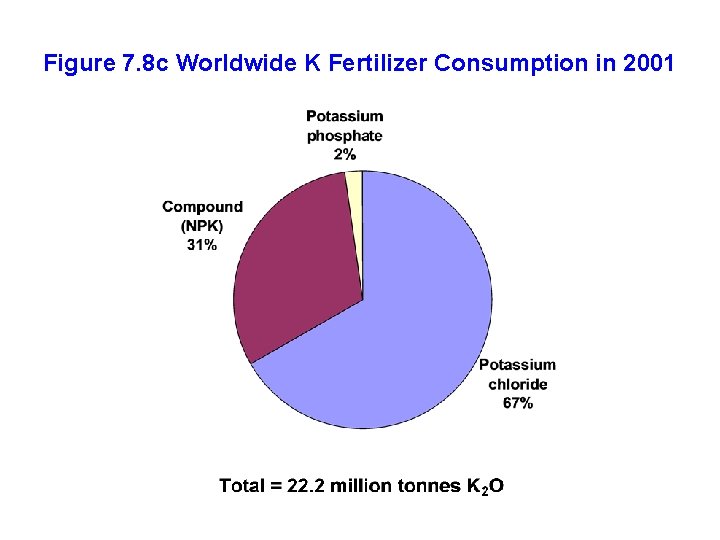
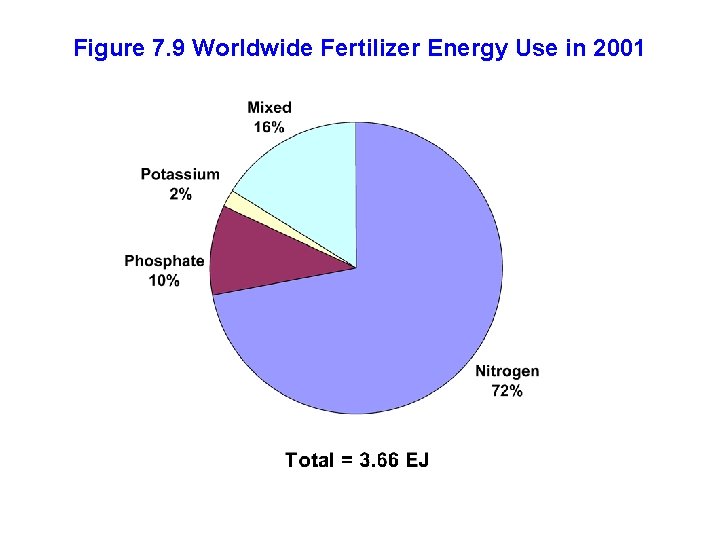
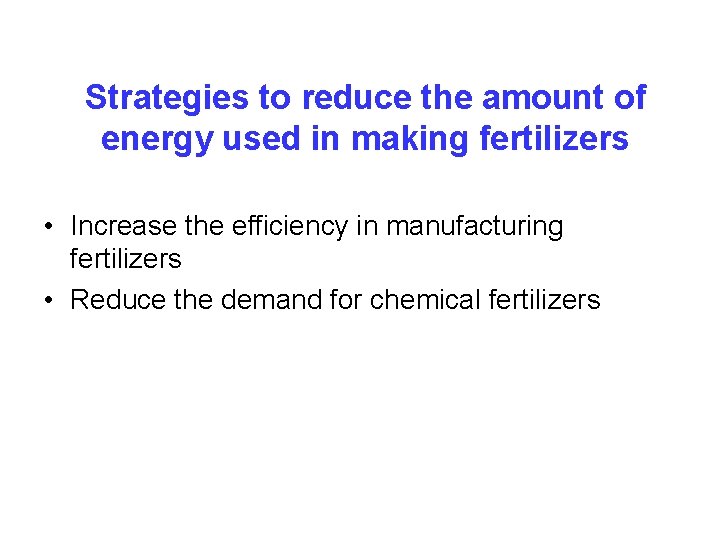
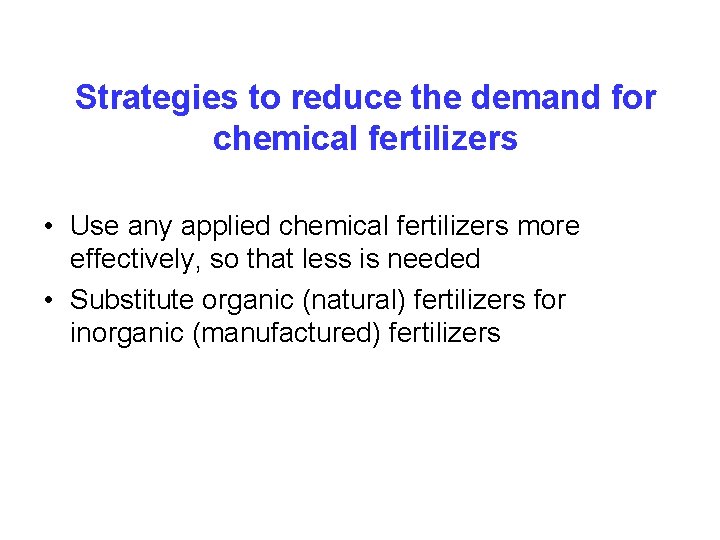
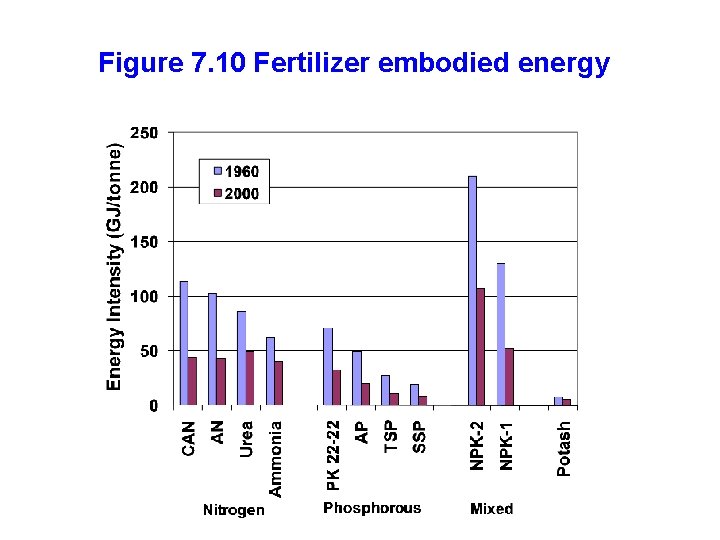
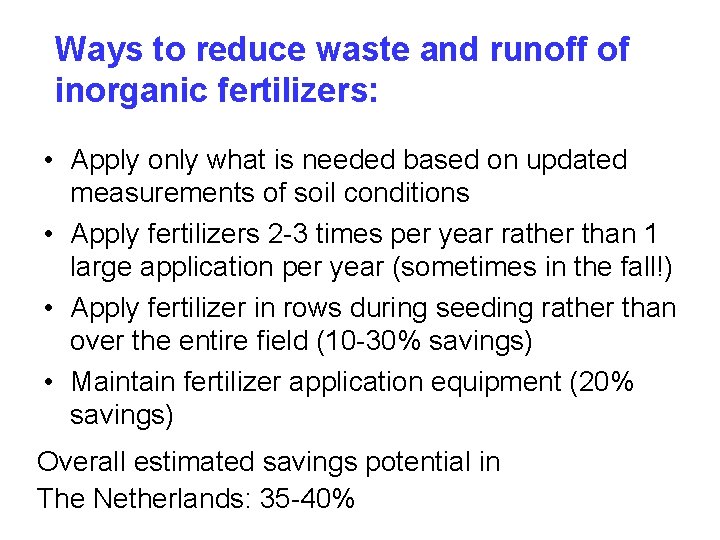
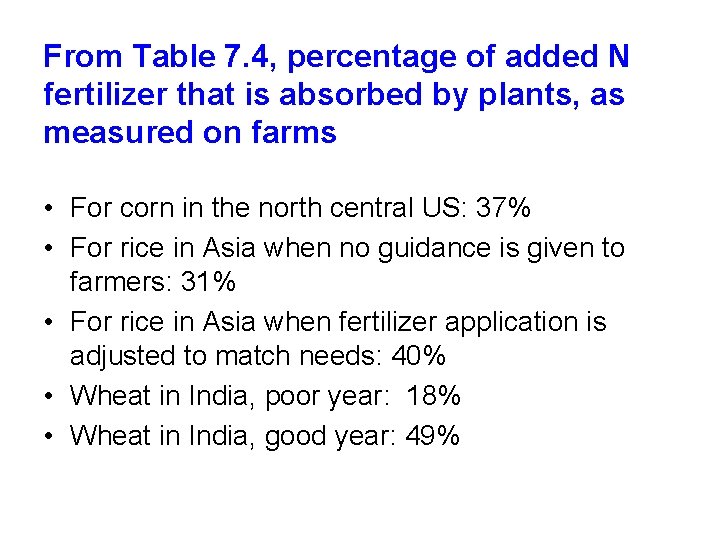
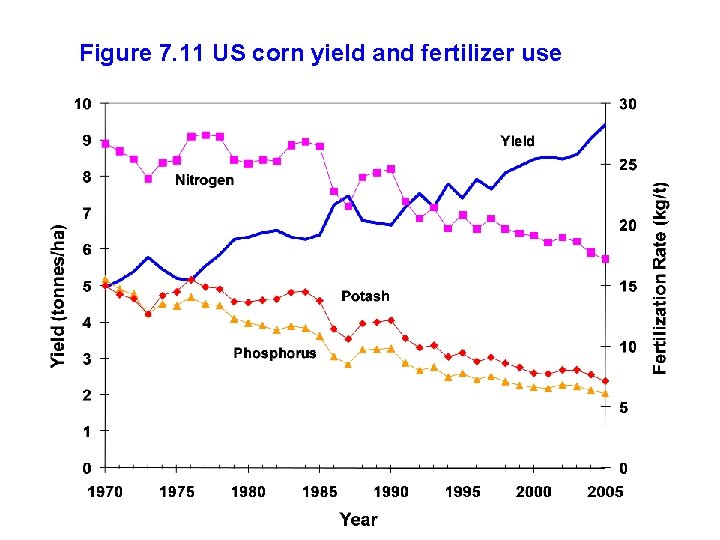
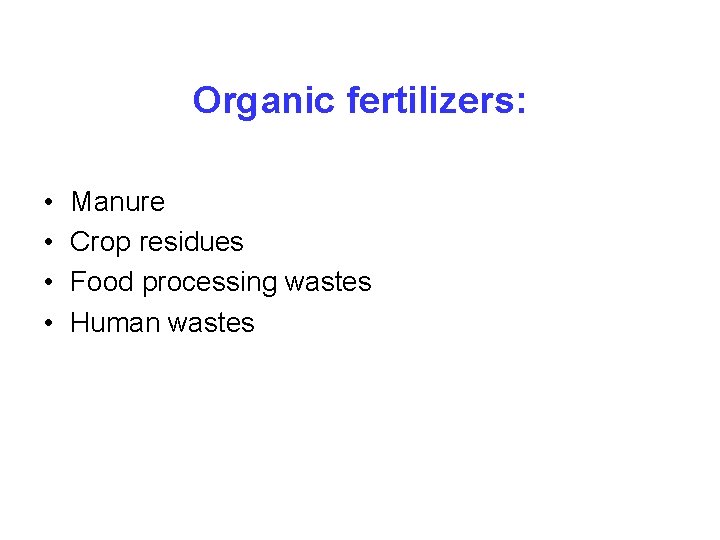
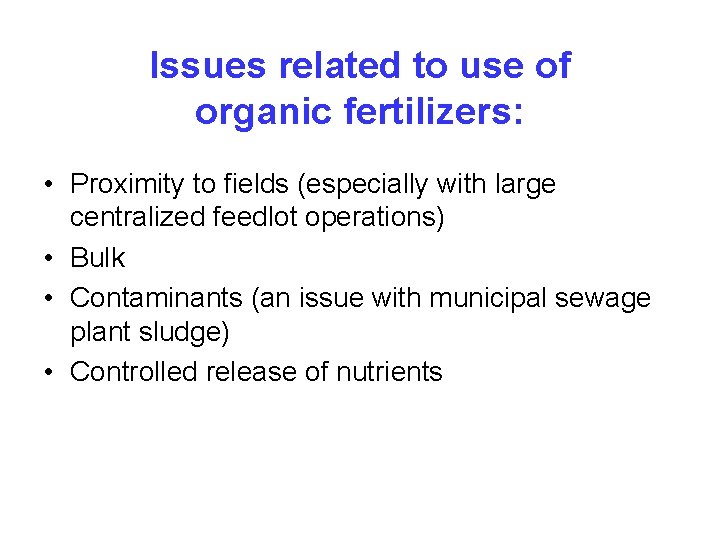
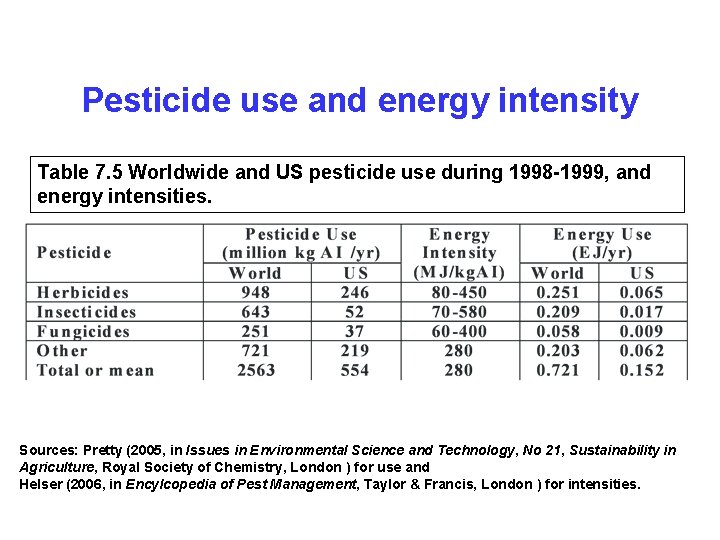
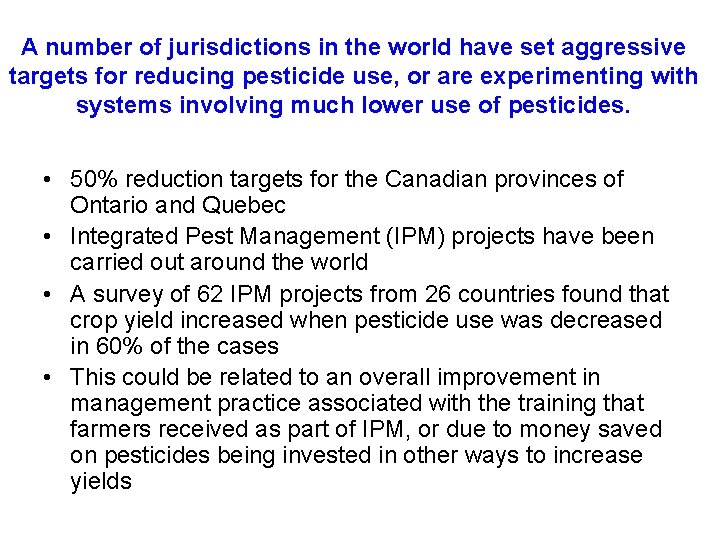
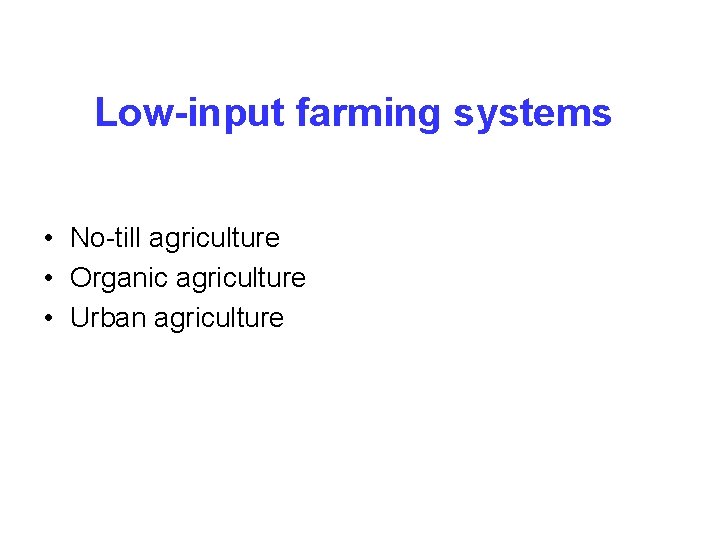
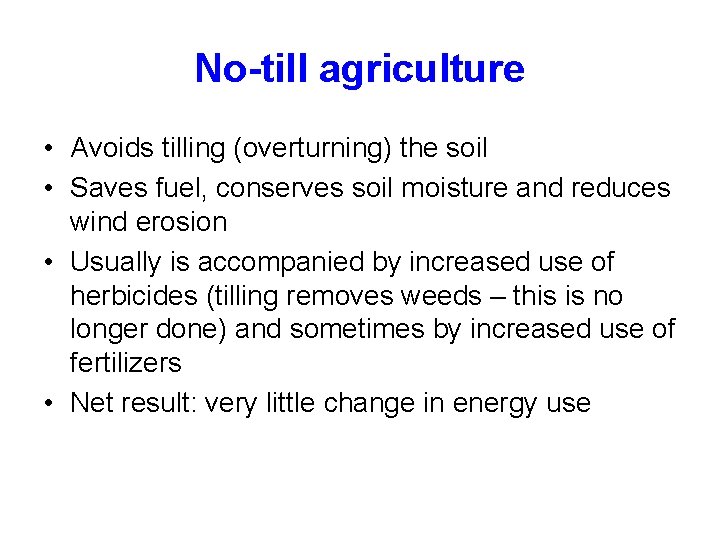
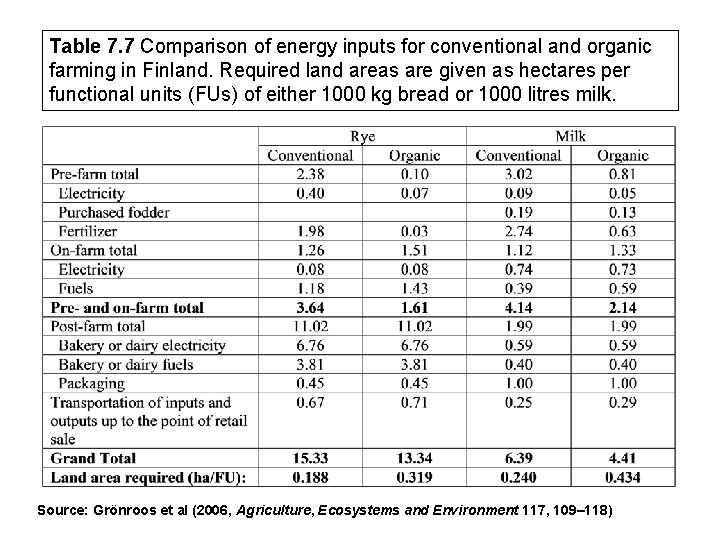
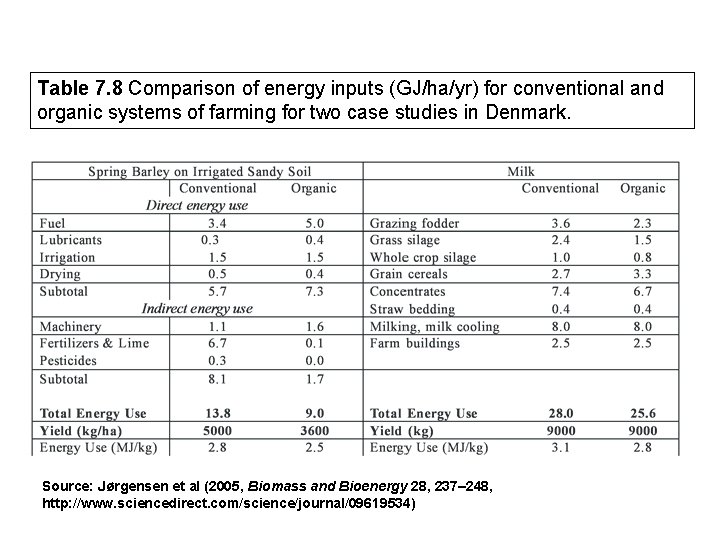
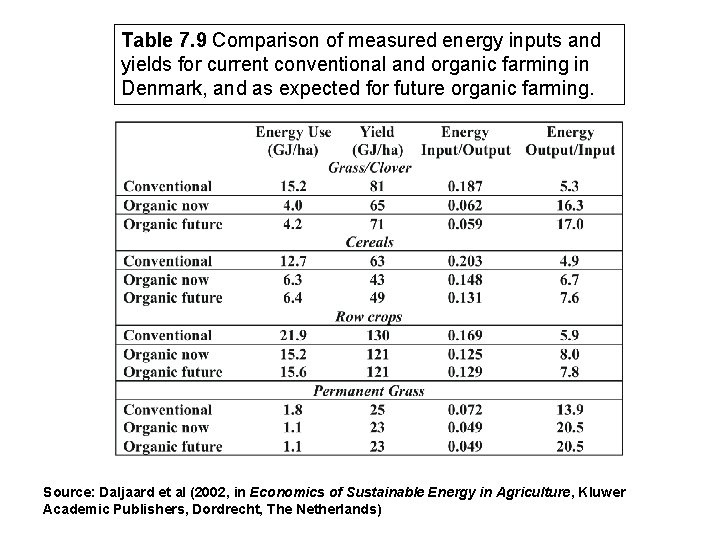
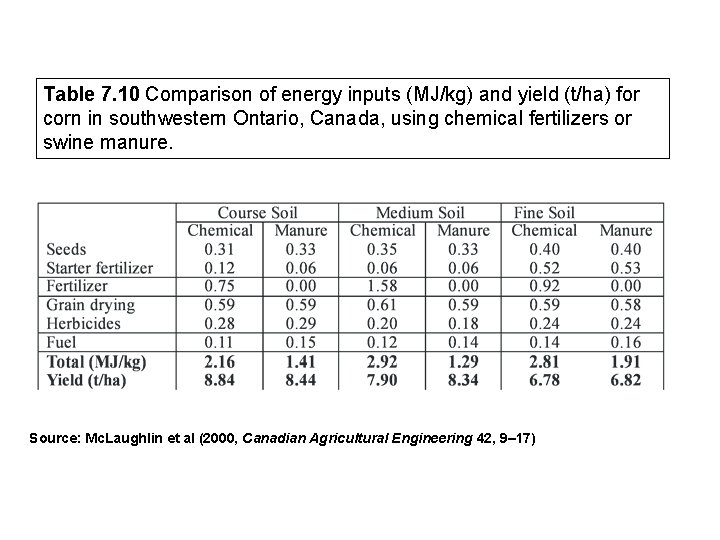
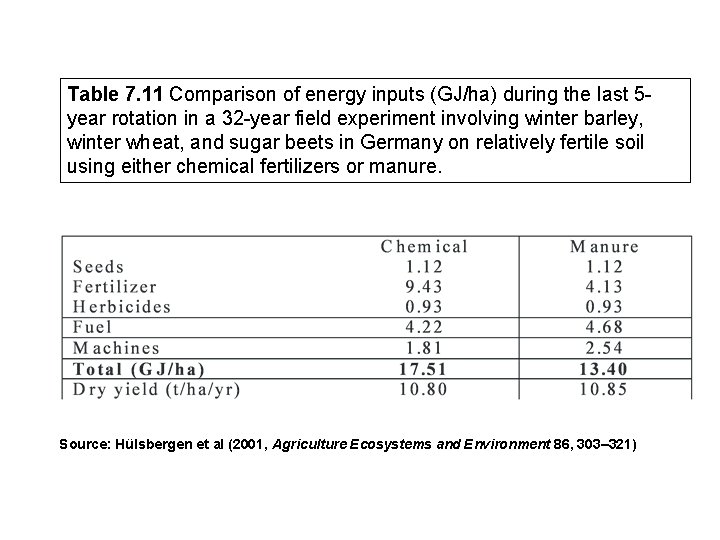
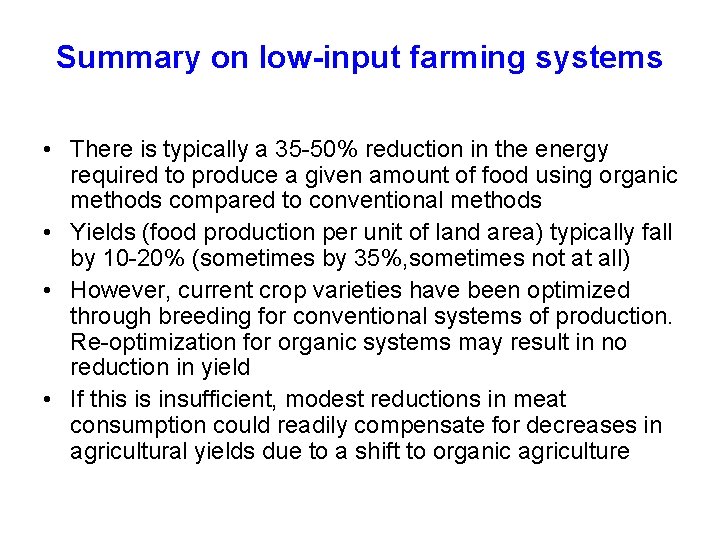
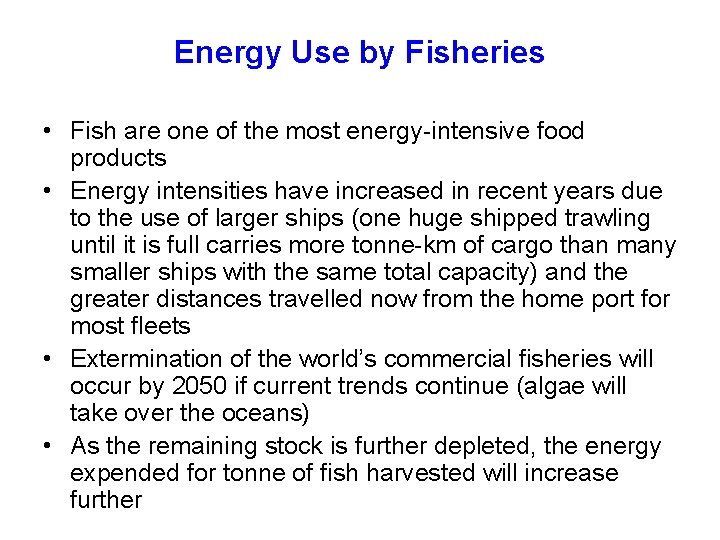
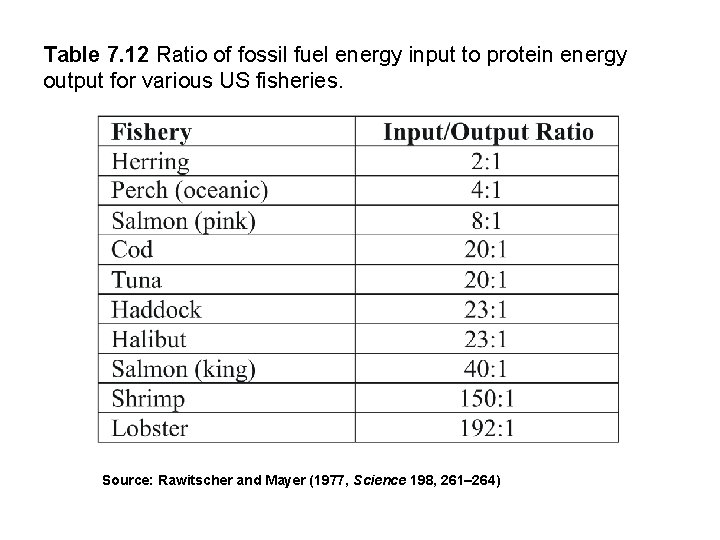
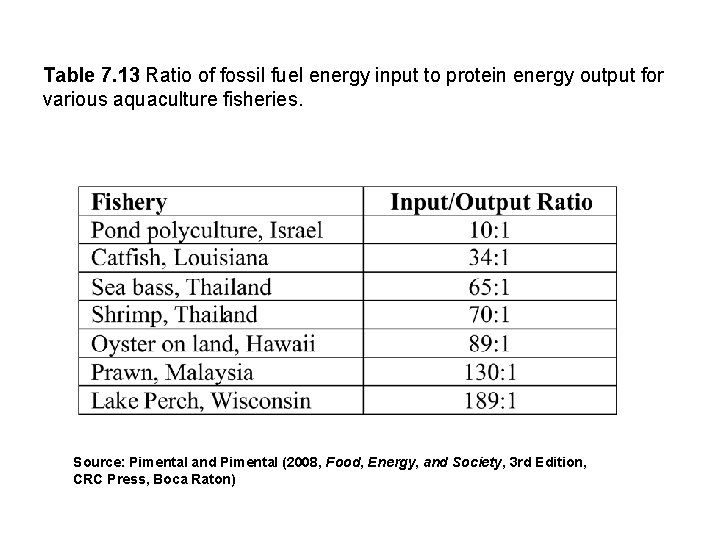
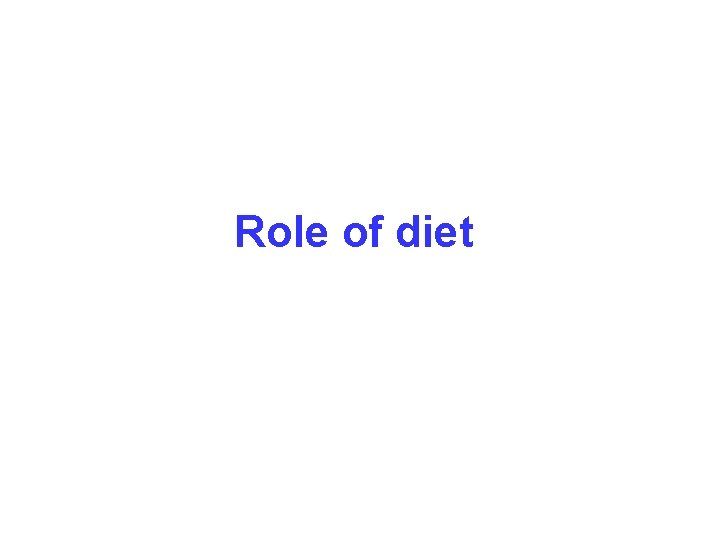
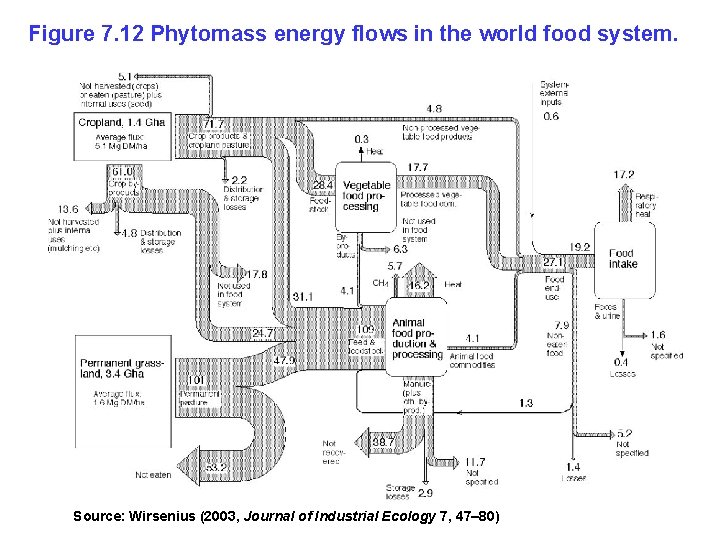
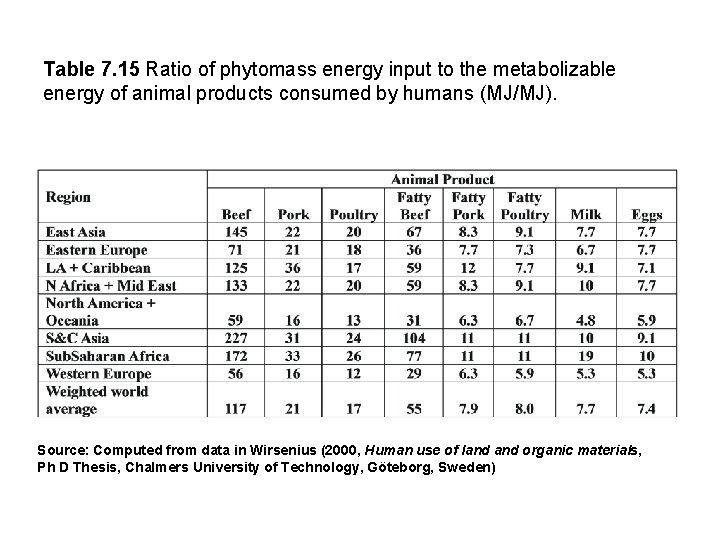
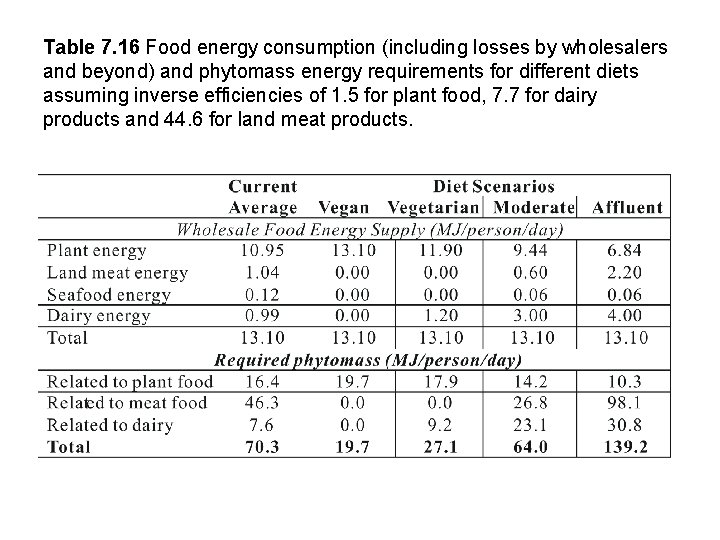
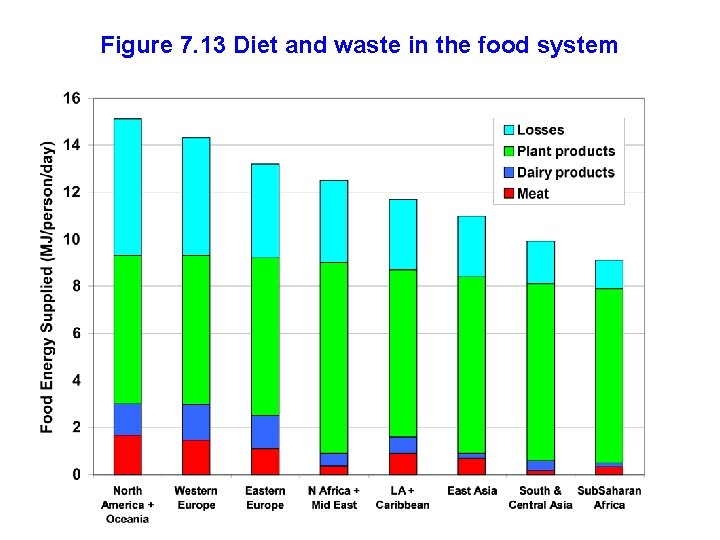
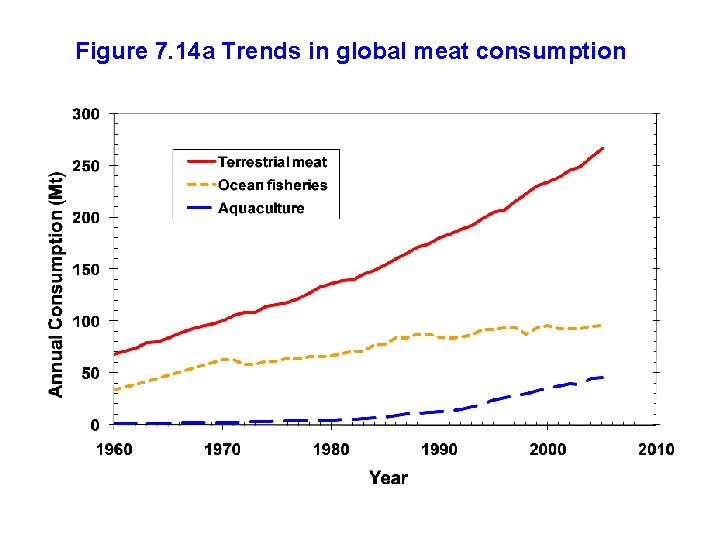
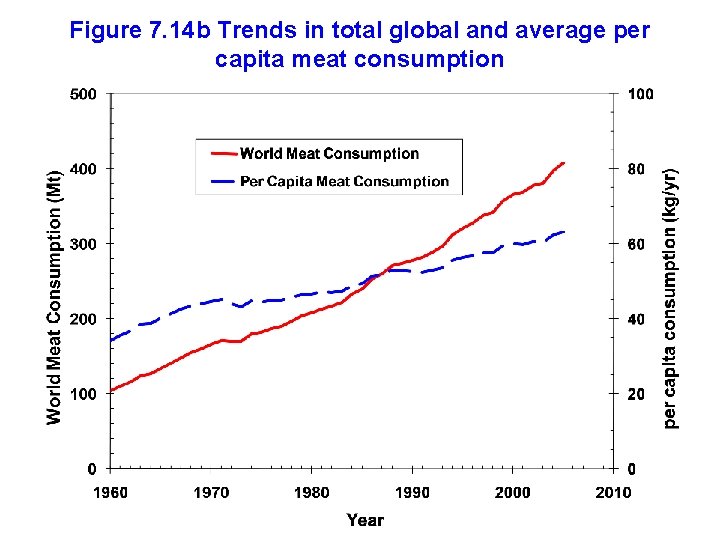
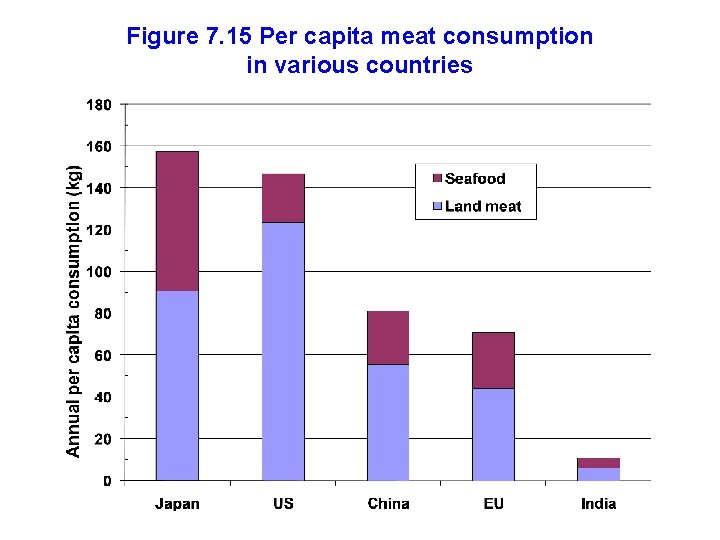
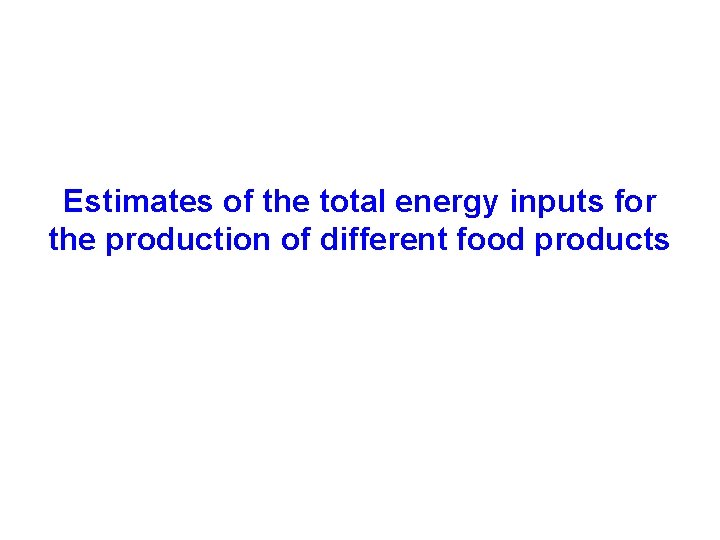
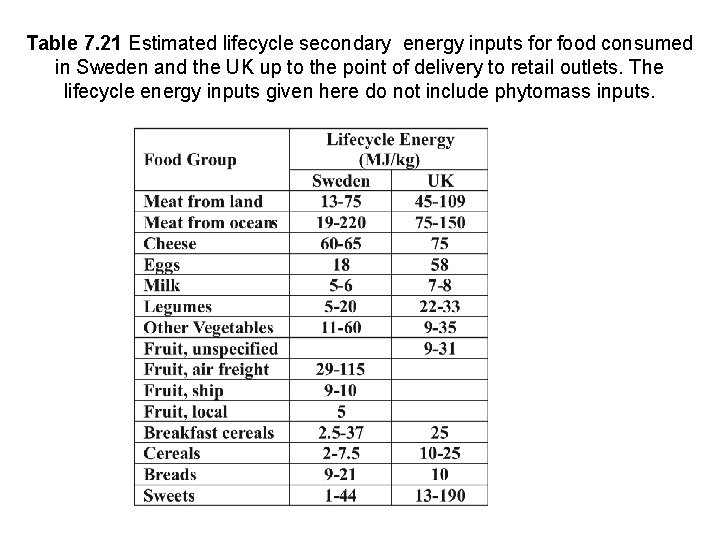
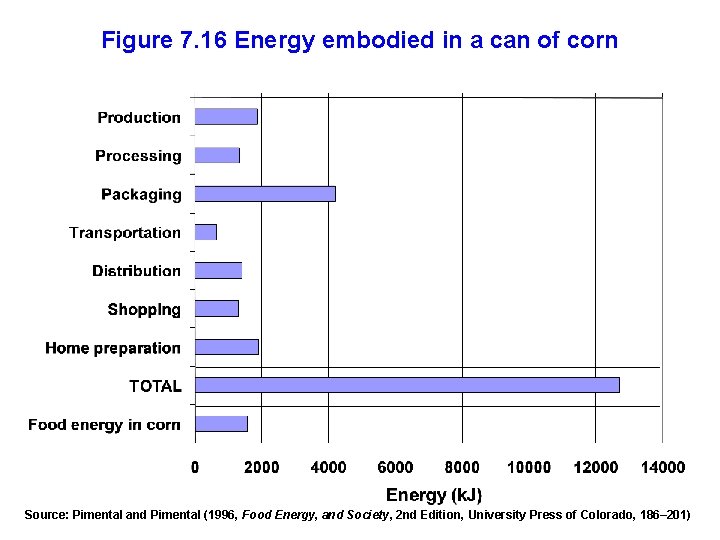
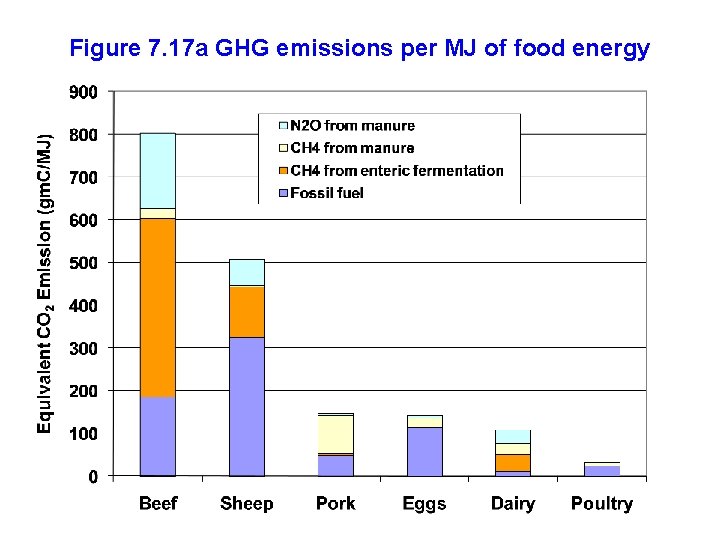
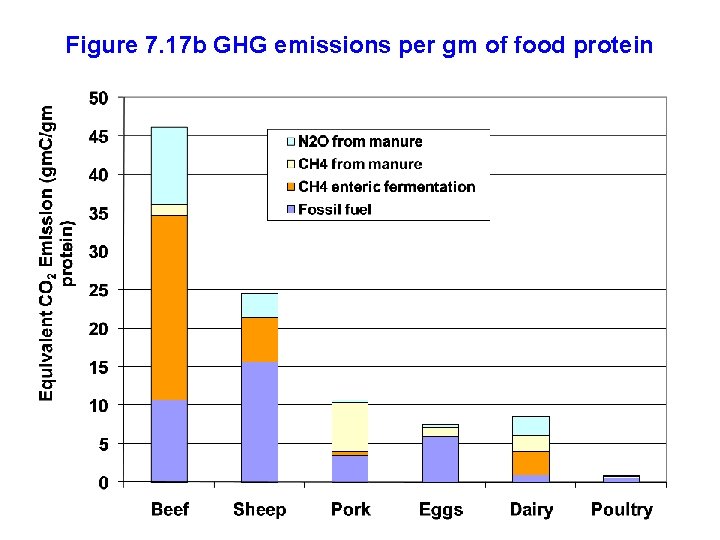
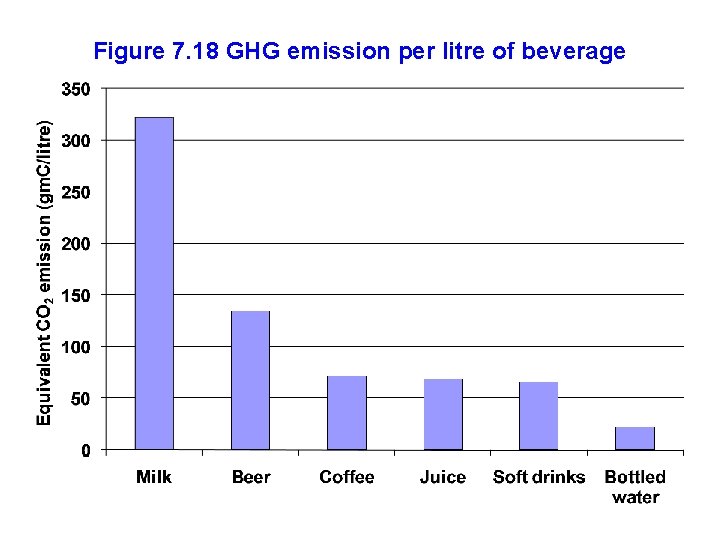
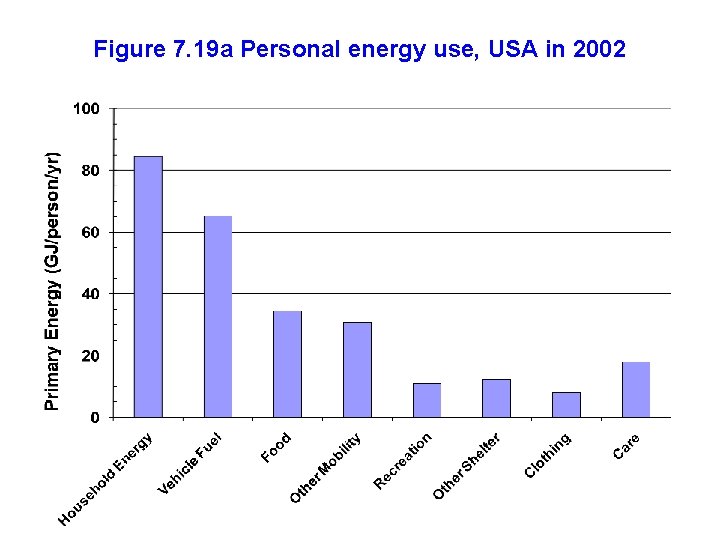
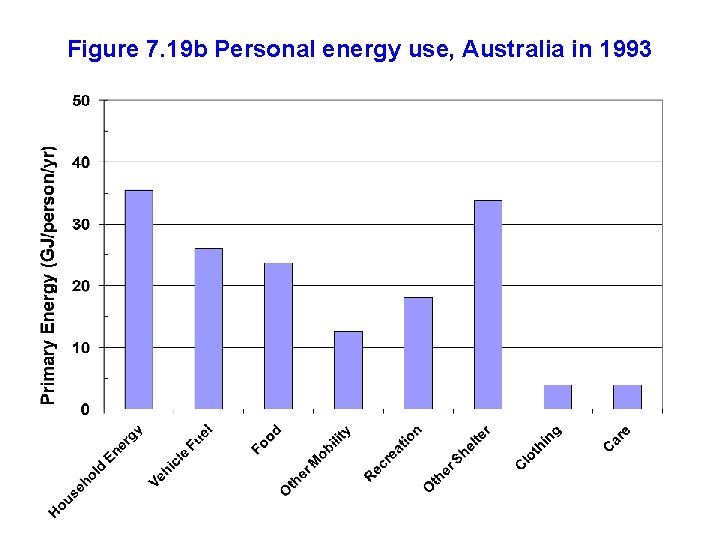
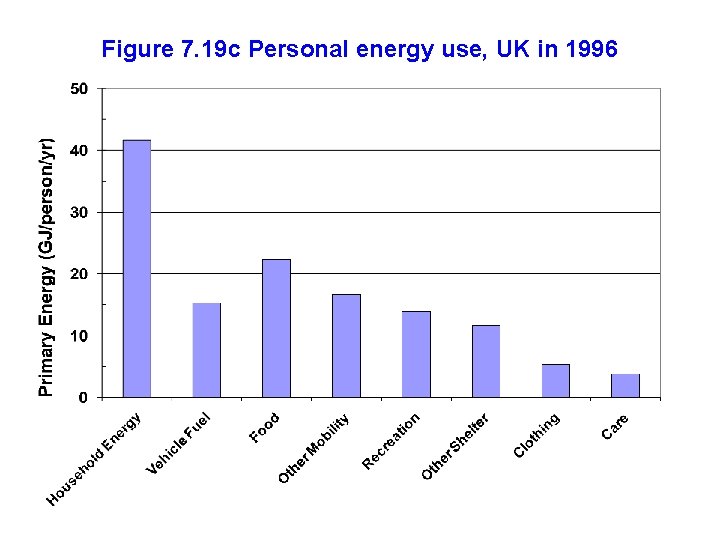
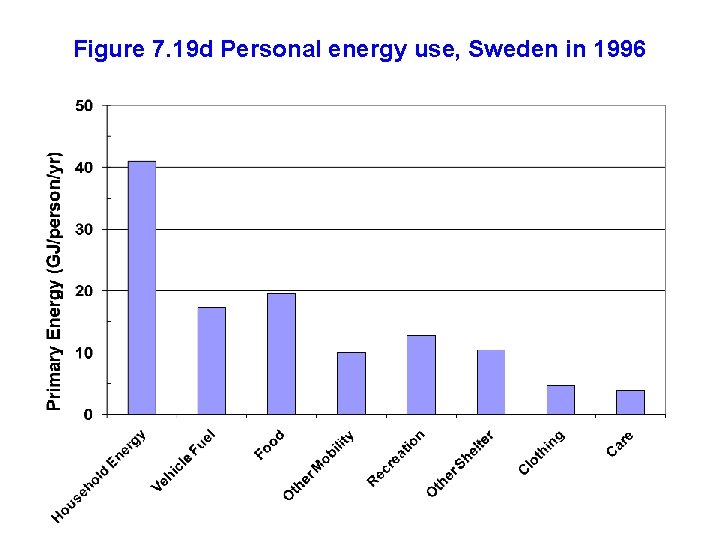
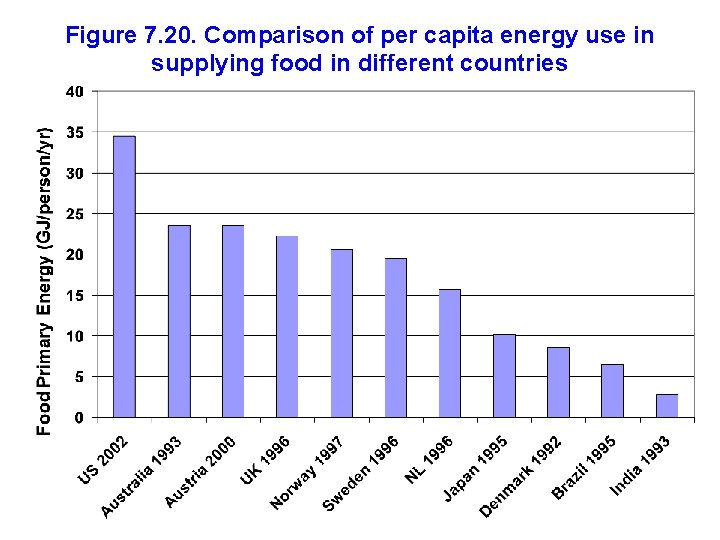
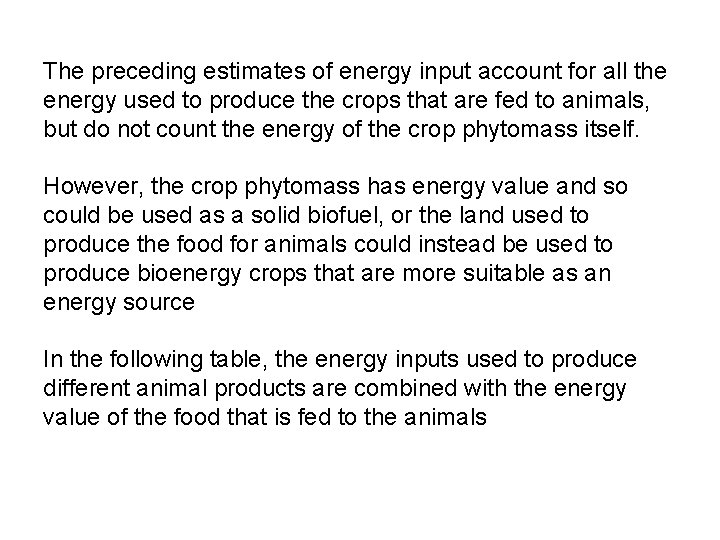
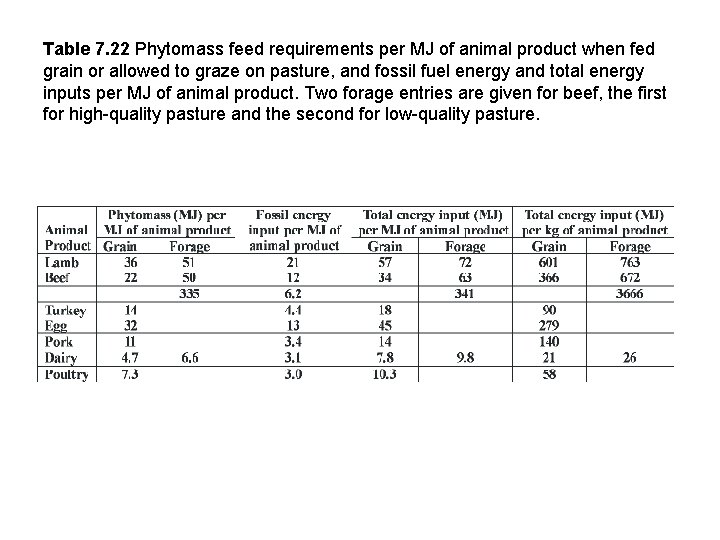
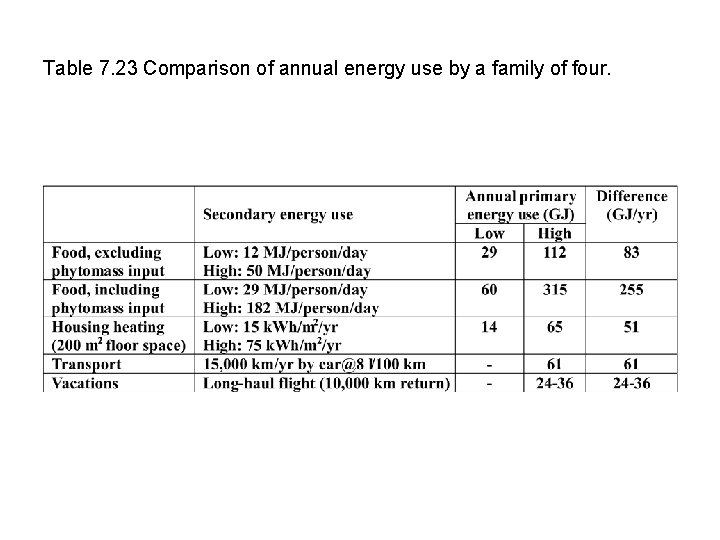
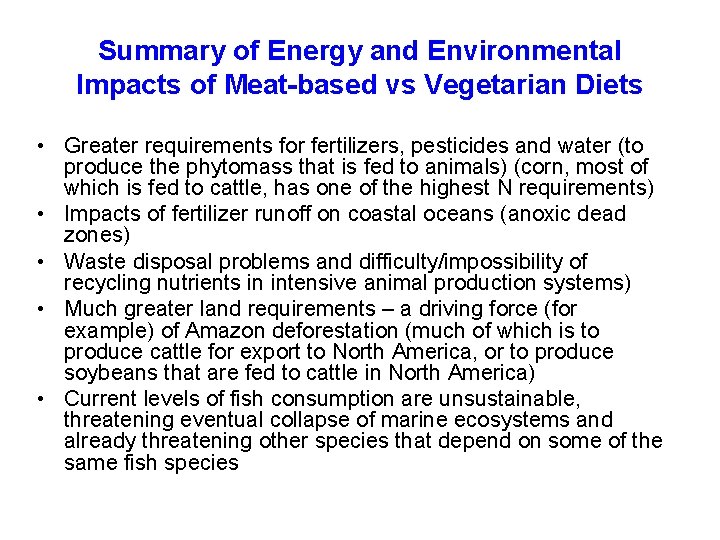
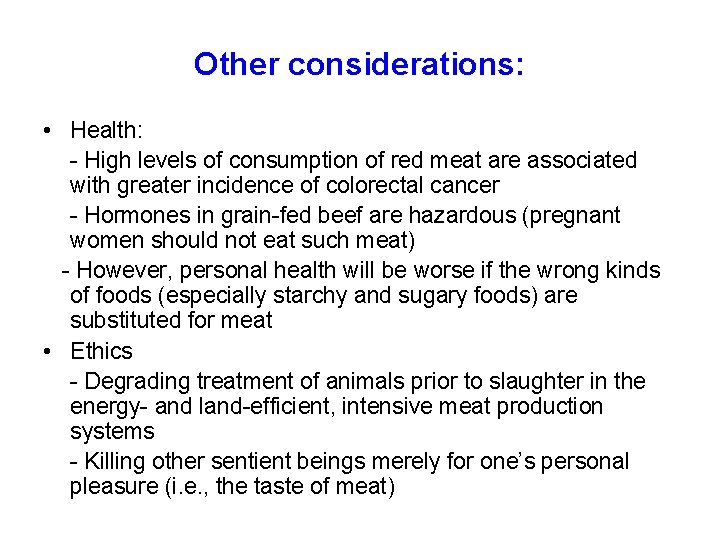
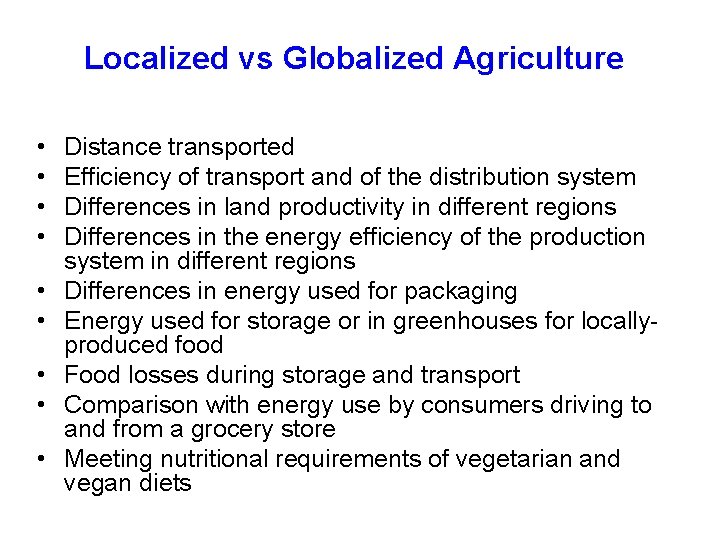
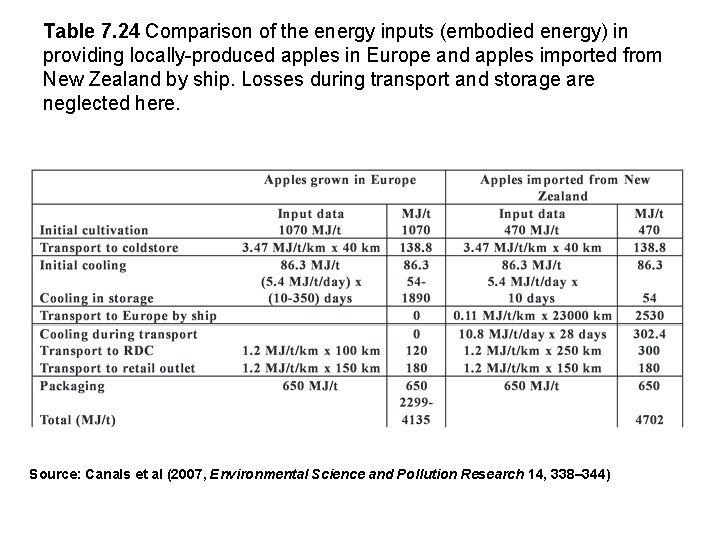
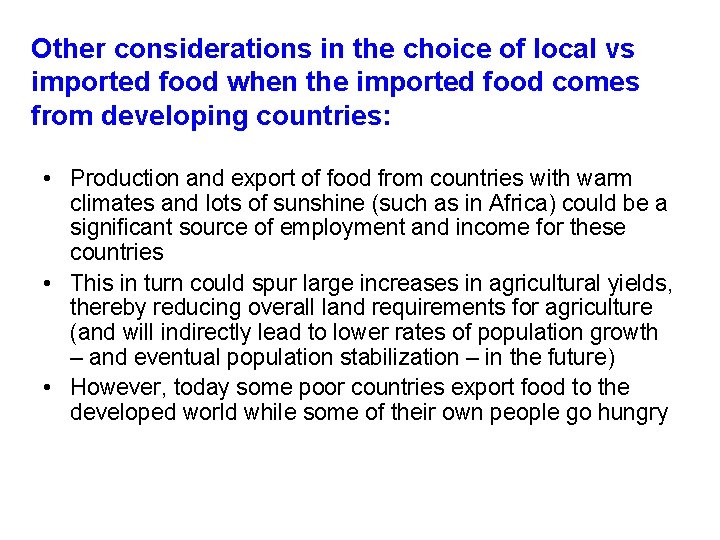
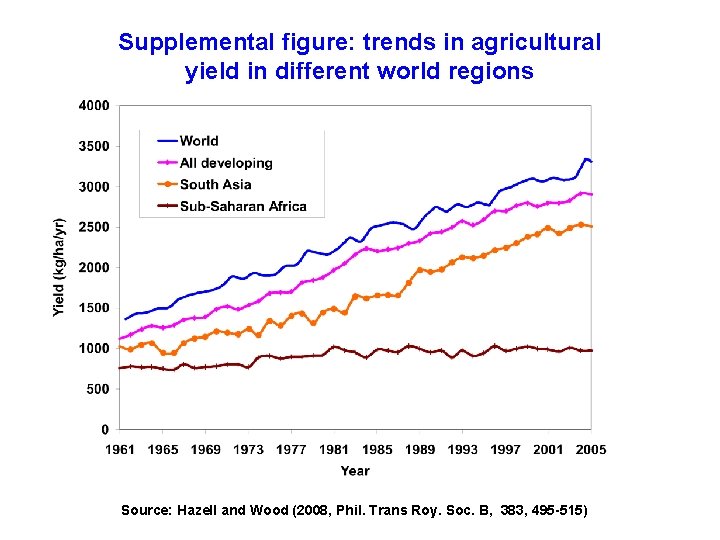
- Slides: 71
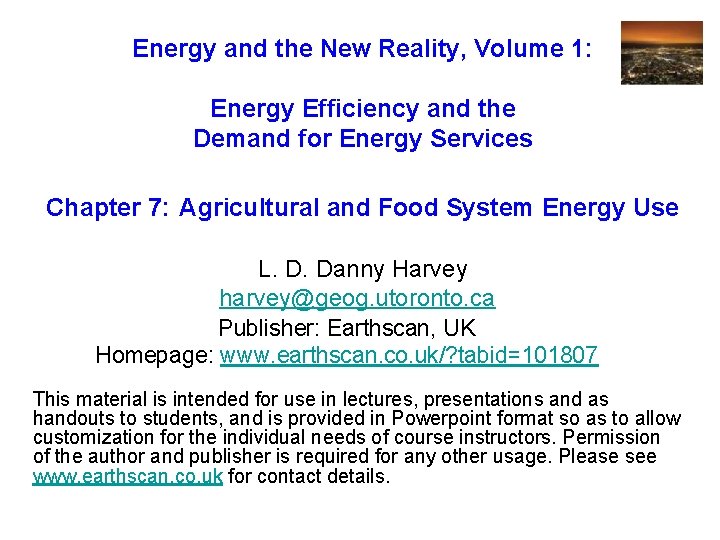
Energy and the New Reality, Volume 1: Energy Efficiency and the Demand for Energy Services Chapter 7: Agricultural and Food System Energy Use L. D. Danny Harvey harvey@geog. utoronto. ca Publisher: Earthscan, UK Homepage: www. earthscan. co. uk/? tabid=101807 This material is intended for use in lectures, presentations and as handouts to students, and is provided in Powerpoint format so as to allow customization for the individual needs of course instructors. Permission of the author and publisher is required for any other usage. Please see www. earthscan. co. uk for contact details.
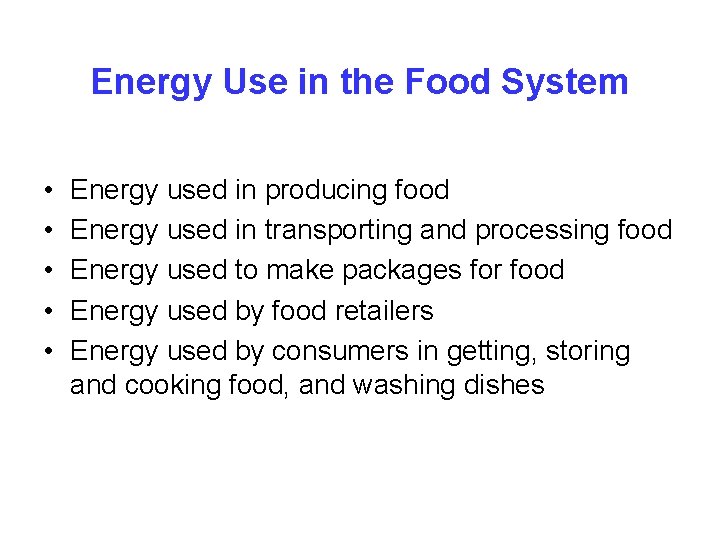
Energy Use in the Food System • • • Energy used in producing food Energy used in transporting and processing food Energy used to make packages for food Energy used by food retailers Energy used by consumers in getting, storing and cooking food, and washing dishes
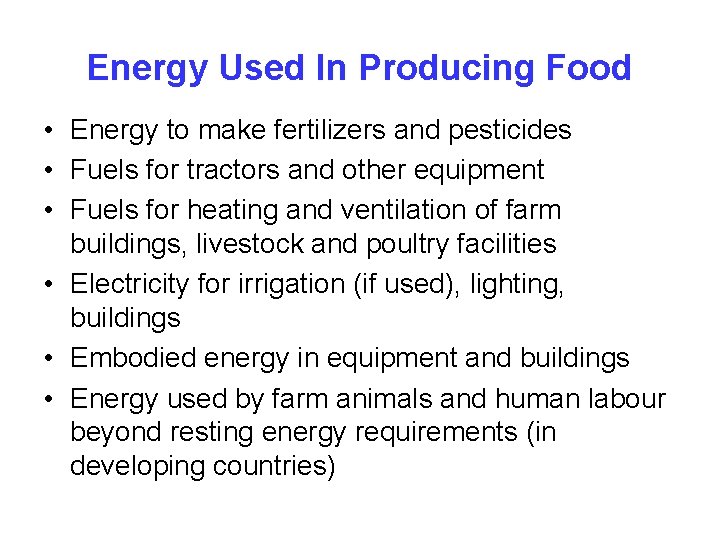
Energy Used In Producing Food • Energy to make fertilizers and pesticides • Fuels for tractors and other equipment • Fuels for heating and ventilation of farm buildings, livestock and poultry facilities • Electricity for irrigation (if used), lighting, buildings • Embodied energy in equipment and buildings • Energy used by farm animals and human labour beyond resting energy requirements (in developing countries)
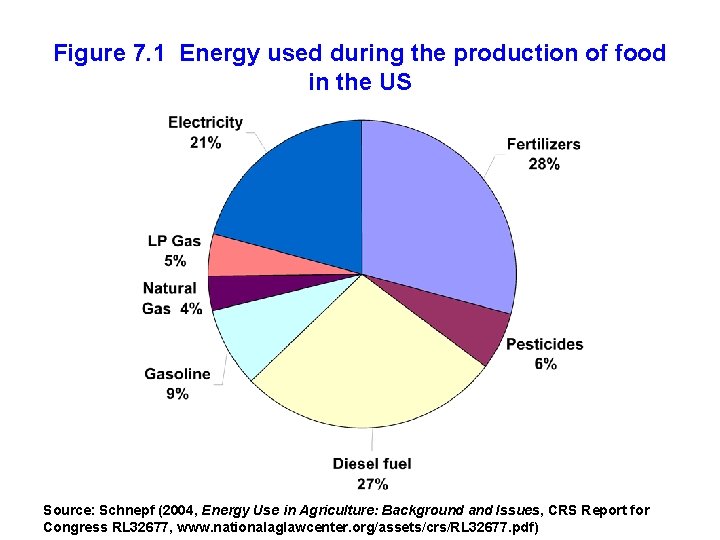
Figure 7. 1 Energy used during the production of food in the US Source: Schnepf (2004, Energy Use in Agriculture: Background and Issues, CRS Report for Congress RL 32677, www. nationalaglawcenter. org/assets/crs/RL 32677. pdf)
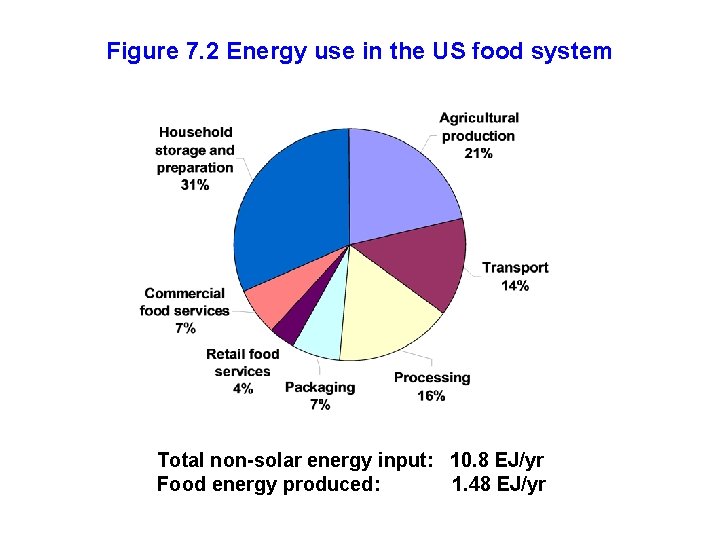
Figure 7. 2 Energy use in the US food system Total non-solar energy input: 10. 8 EJ/yr Food energy produced: 1. 48 EJ/yr
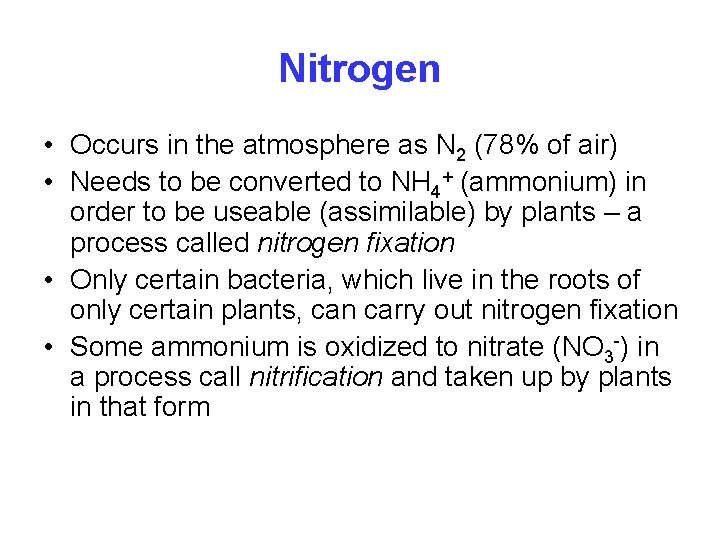
Nitrogen • Occurs in the atmosphere as N 2 (78% of air) • Needs to be converted to NH 4+ (ammonium) in order to be useable (assimilable) by plants – a process called nitrogen fixation • Only certain bacteria, which live in the roots of only certain plants, can carry out nitrogen fixation • Some ammonium is oxidized to nitrate (NO 3 -) in a process call nitrification and taken up by plants in that form
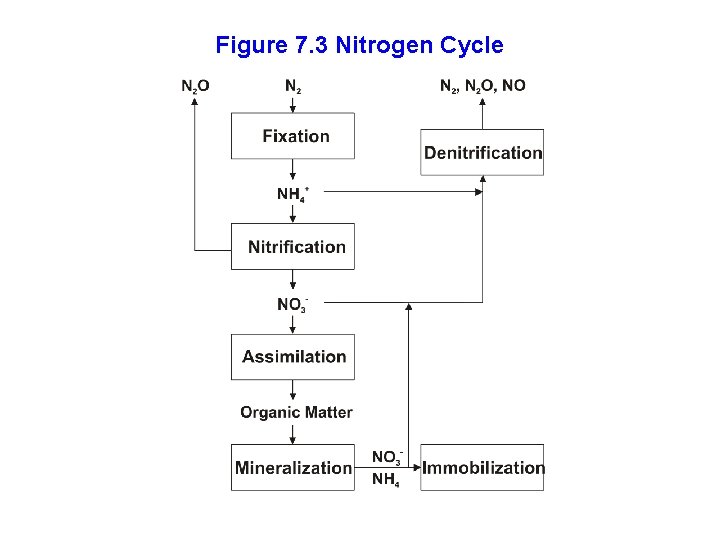
Figure 7. 3 Nitrogen Cycle
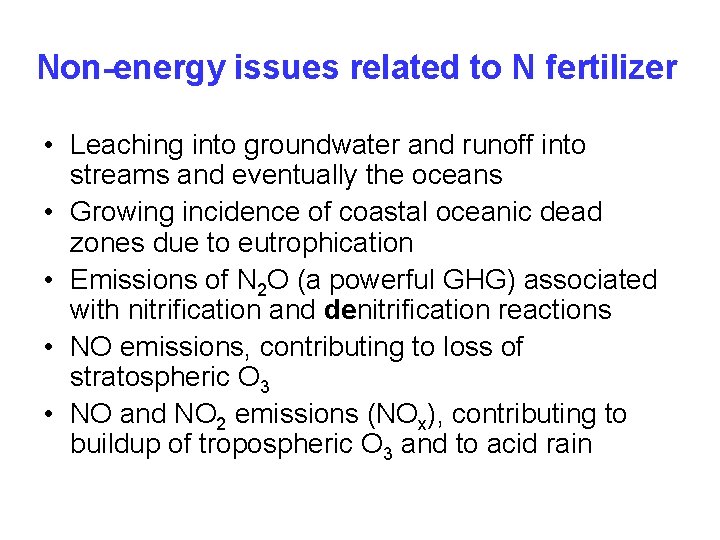
Non-energy issues related to N fertilizer • Leaching into groundwater and runoff into streams and eventually the oceans • Growing incidence of coastal oceanic dead zones due to eutrophication • Emissions of N 2 O (a powerful GHG) associated with nitrification and denitrification reactions • NO emissions, contributing to loss of stratospheric O 3 • NO and NO 2 emissions (NOx), contributing to buildup of tropospheric O 3 and to acid rain
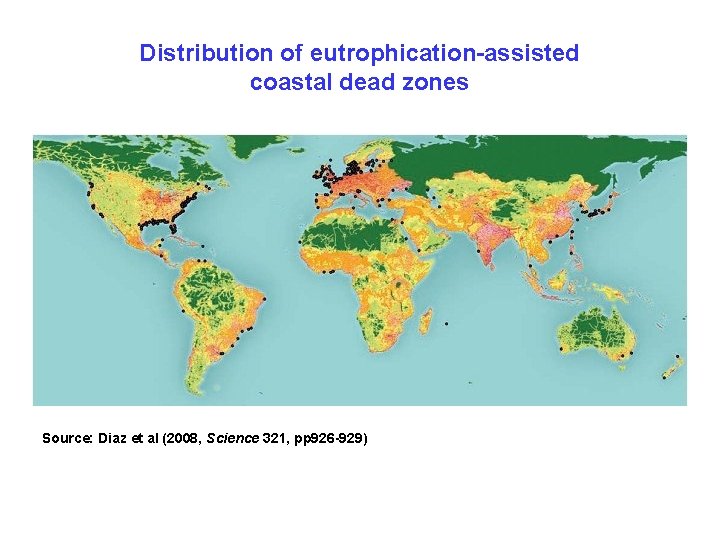
Distribution of eutrophication-assisted coastal dead zones Source: Diaz et al (2008, Science 321, pp 926 -929)
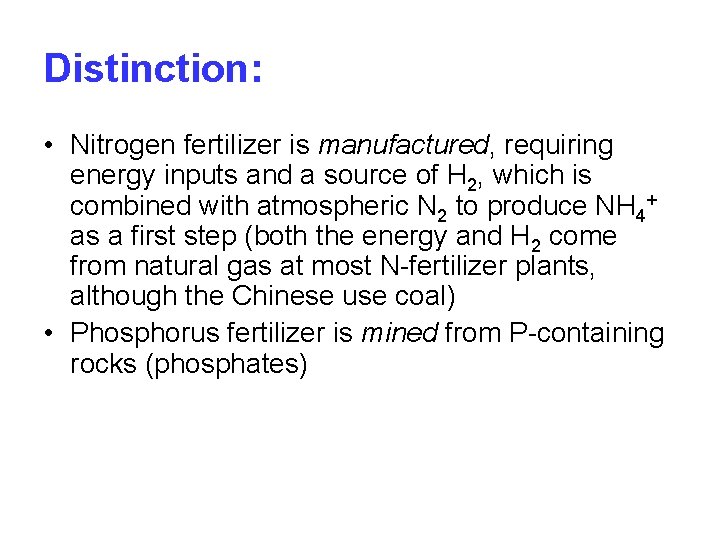
Distinction: • Nitrogen fertilizer is manufactured, requiring energy inputs and a source of H 2, which is combined with atmospheric N 2 to produce NH 4+ as a first step (both the energy and H 2 come from natural gas at most N-fertilizer plants, although the Chinese use coal) • Phosphorus fertilizer is mined from P-containing rocks (phosphates)
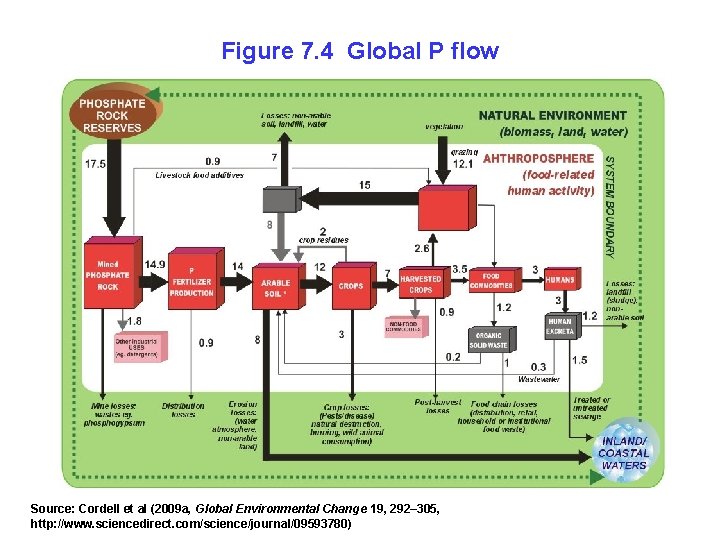
Figure 7. 4 Global P flow Source: Cordell et al (2009 a, Global Environmental Change 19, 292– 305, http: //www. sciencedirect. com/science/journal/09593780)
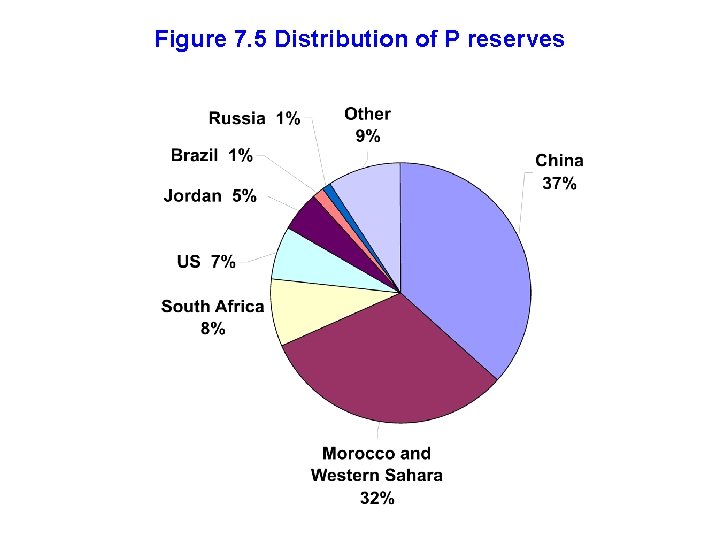
Figure 7. 5 Distribution of P reserves
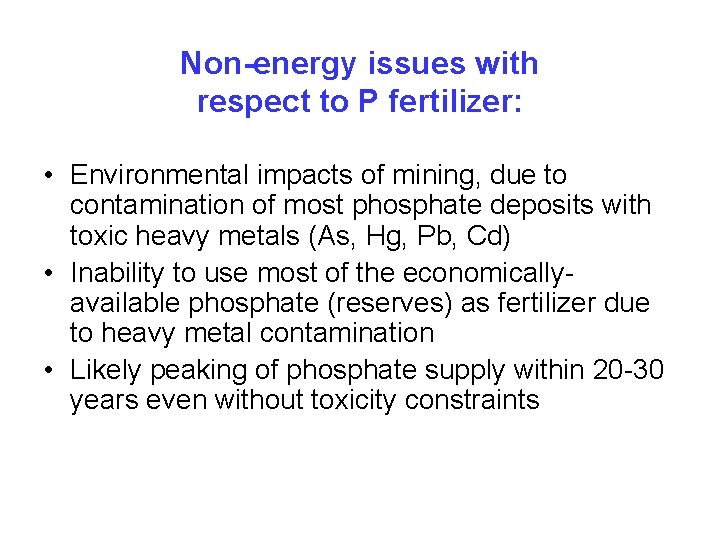
Non-energy issues with respect to P fertilizer: • Environmental impacts of mining, due to contamination of most phosphate deposits with toxic heavy metals (As, Hg, Pb, Cd) • Inability to use most of the economicallyavailable phosphate (reserves) as fertilizer due to heavy metal contamination • Likely peaking of phosphate supply within 20 -30 years even without toxicity constraints
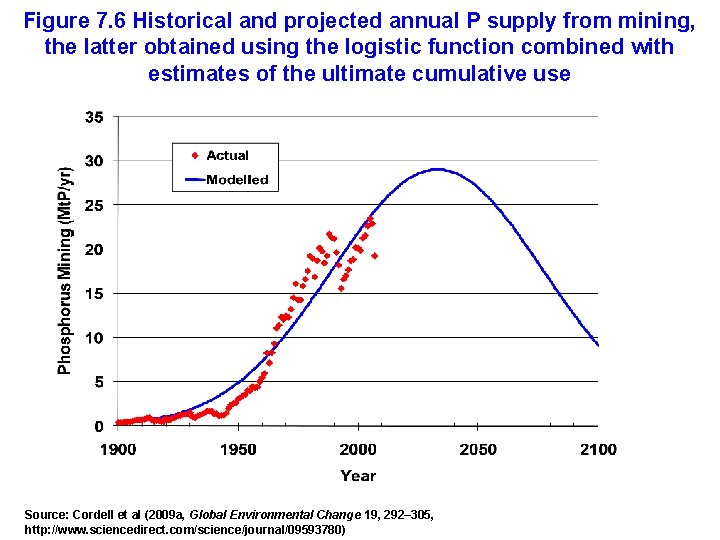
Figure 7. 6 Historical and projected annual P supply from mining, the latter obtained using the logistic function combined with estimates of the ultimate cumulative use Source: Cordell et al (2009 a, Global Environmental Change 19, 292– 305, http: //www. sciencedirect. com/science/journal/09593780)
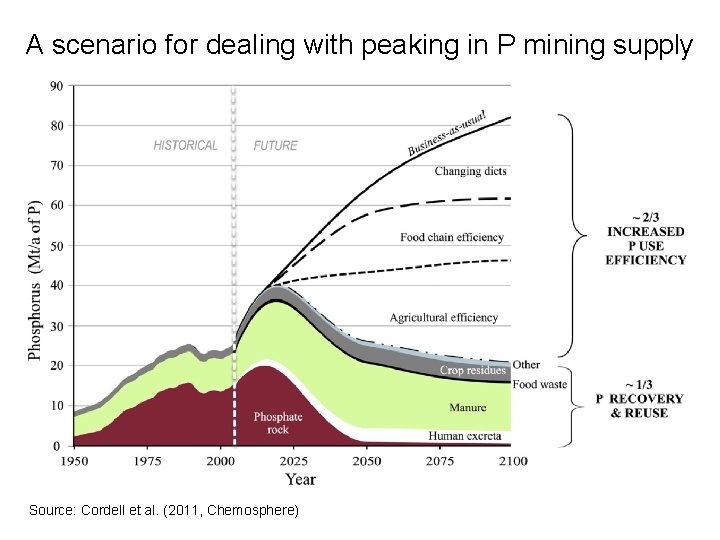
A scenario for dealing with peaking in P mining supply Source: Cordell et al. (2011, Chemosphere)
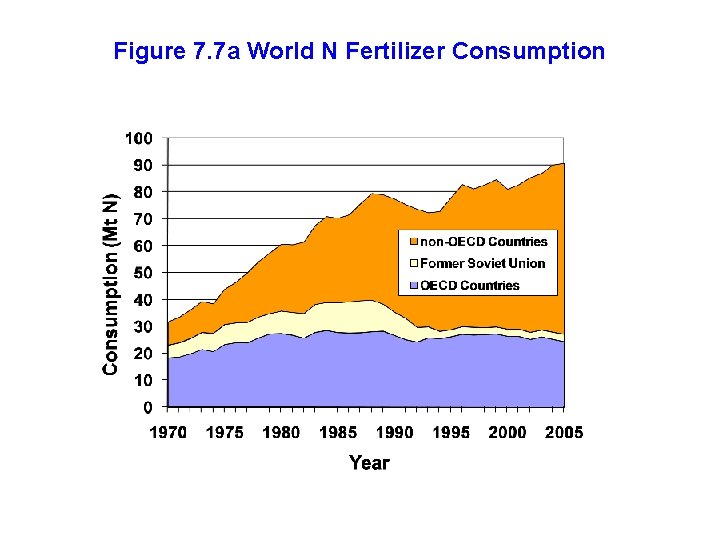
Figure 7. 7 a World N Fertilizer Consumption
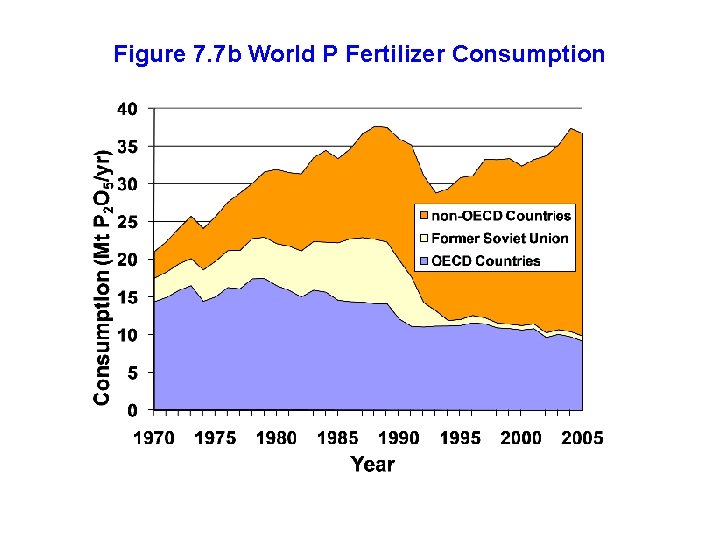
Figure 7. 7 b World P Fertilizer Consumption
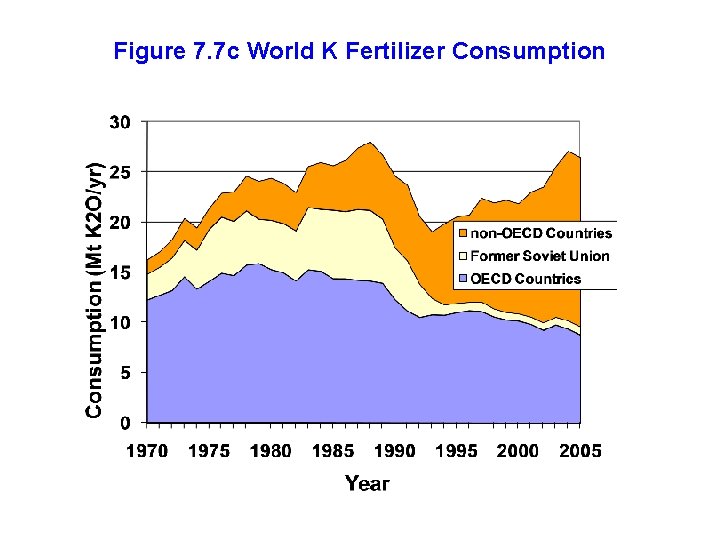
Figure 7. 7 c World K Fertilizer Consumption
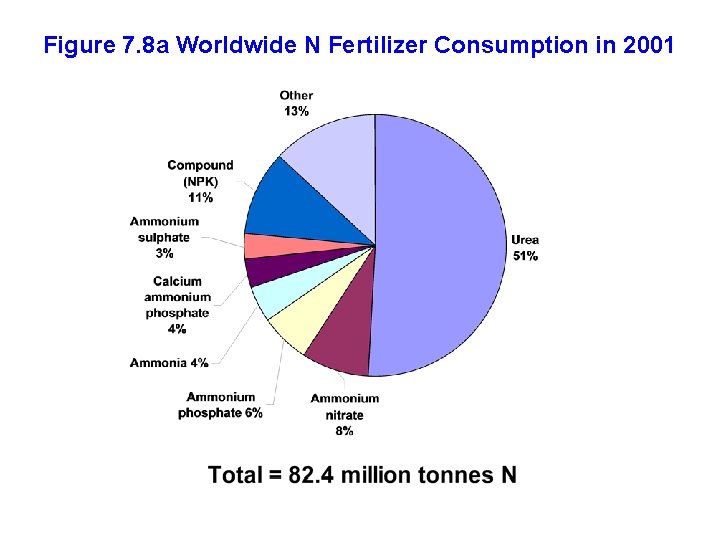
Figure 7. 8 a Worldwide N Fertilizer Consumption in 2001
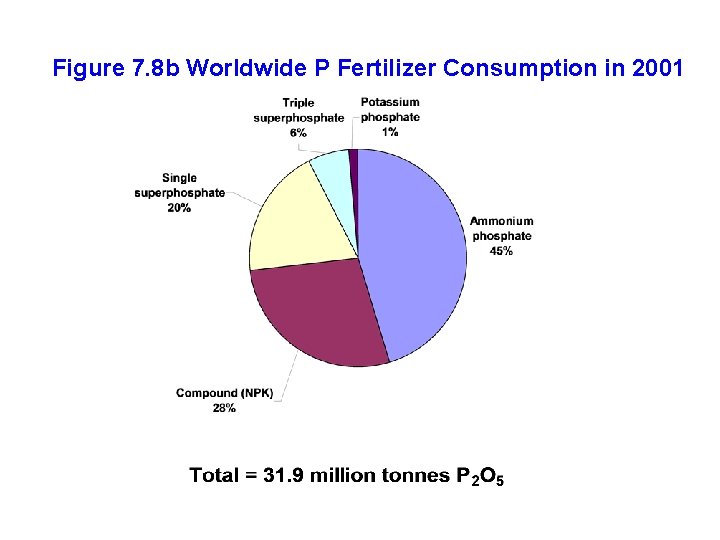
Figure 7. 8 b Worldwide P Fertilizer Consumption in 2001
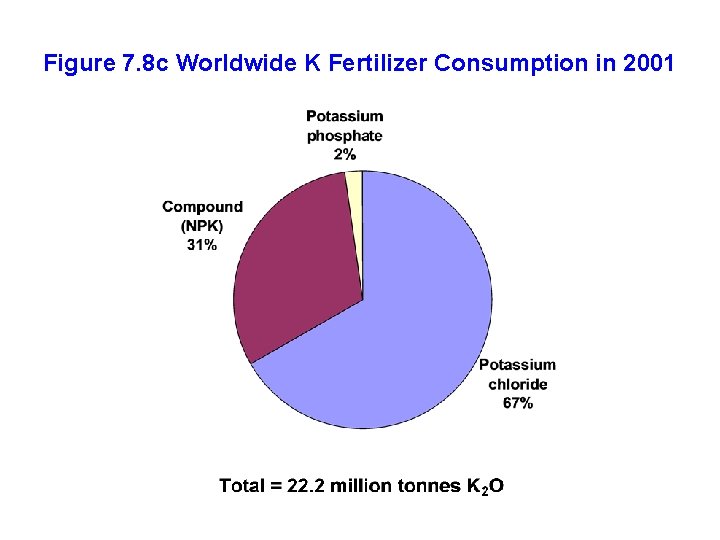
Figure 7. 8 c Worldwide K Fertilizer Consumption in 2001
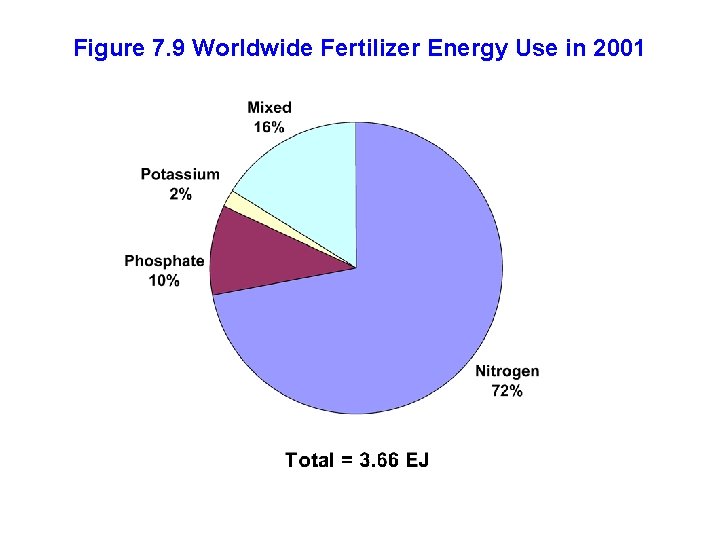
Figure 7. 9 Worldwide Fertilizer Energy Use in 2001
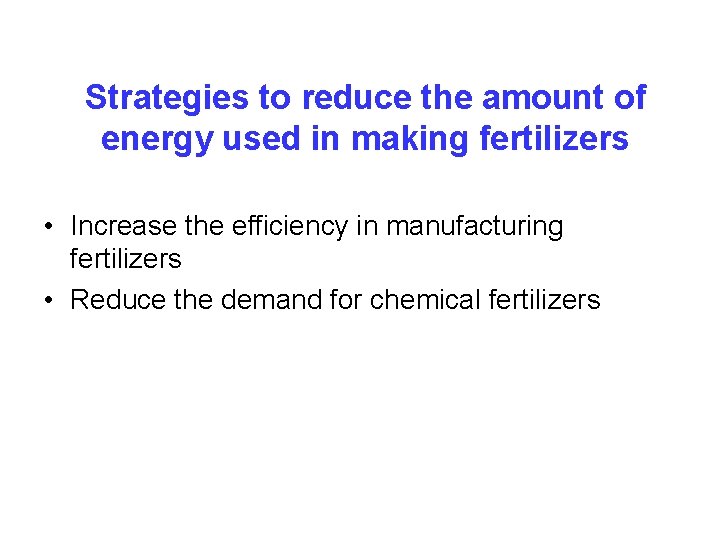
Strategies to reduce the amount of energy used in making fertilizers • Increase the efficiency in manufacturing fertilizers • Reduce the demand for chemical fertilizers
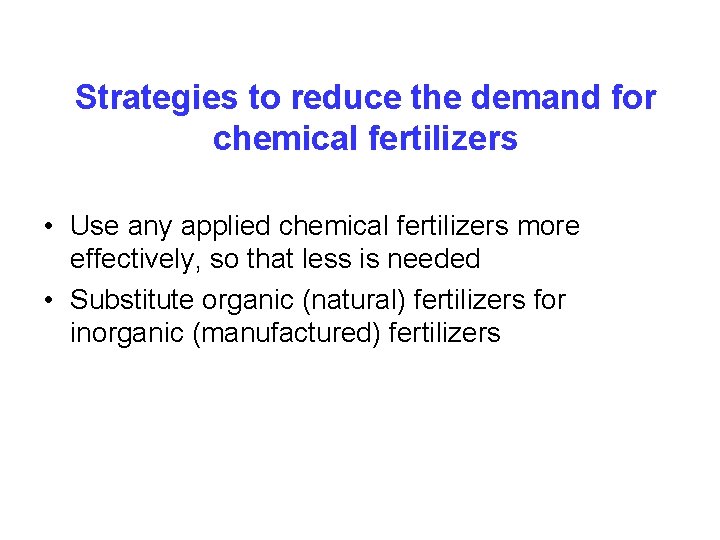
Strategies to reduce the demand for chemical fertilizers • Use any applied chemical fertilizers more effectively, so that less is needed • Substitute organic (natural) fertilizers for inorganic (manufactured) fertilizers
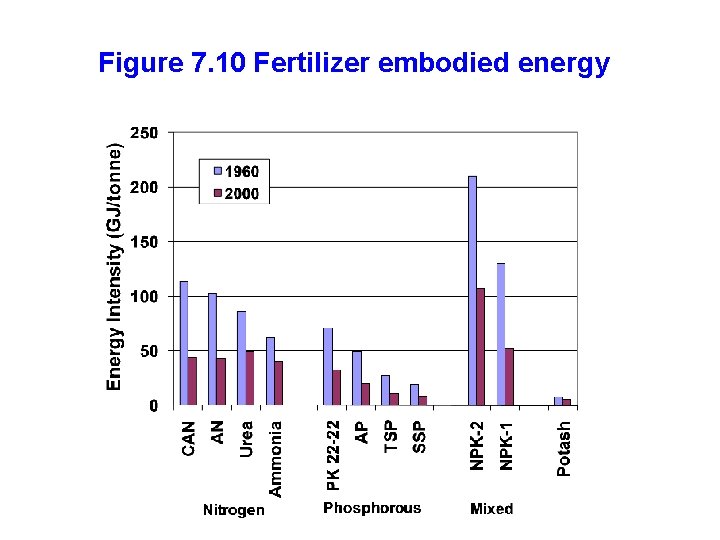
Figure 7. 10 Fertilizer embodied energy
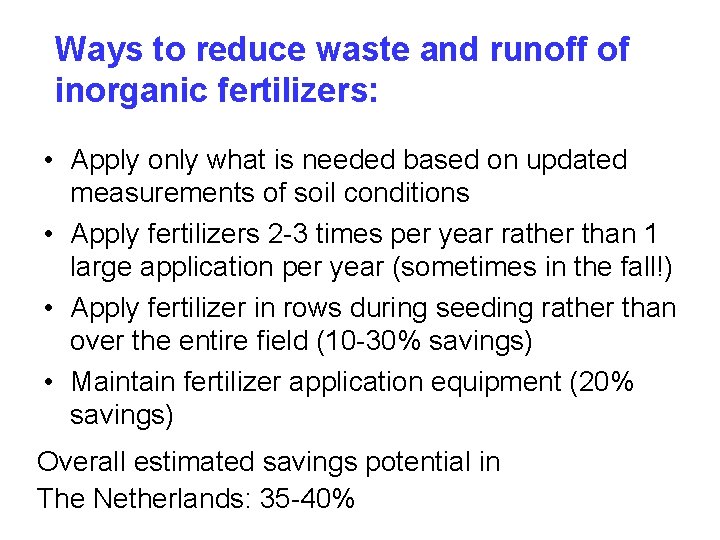
Ways to reduce waste and runoff of inorganic fertilizers: • Apply only what is needed based on updated measurements of soil conditions • Apply fertilizers 2 -3 times per year rather than 1 large application per year (sometimes in the fall!) • Apply fertilizer in rows during seeding rather than over the entire field (10 -30% savings) • Maintain fertilizer application equipment (20% savings) Overall estimated savings potential in The Netherlands: 35 -40%
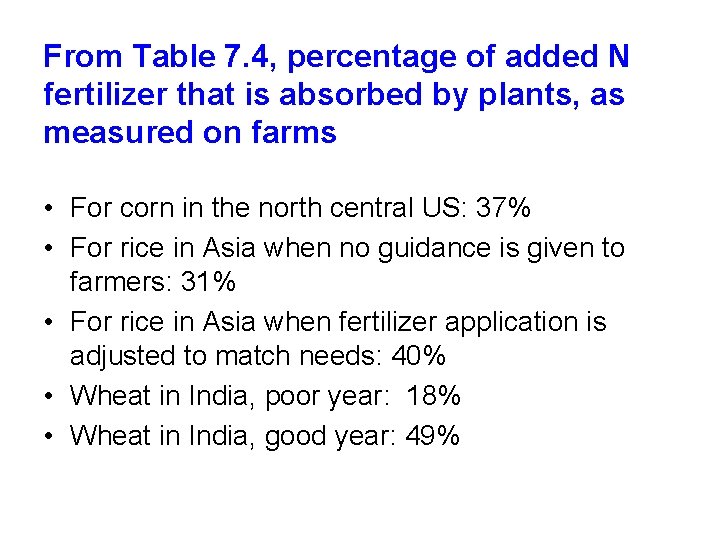
From Table 7. 4, percentage of added N fertilizer that is absorbed by plants, as measured on farms • For corn in the north central US: 37% • For rice in Asia when no guidance is given to farmers: 31% • For rice in Asia when fertilizer application is adjusted to match needs: 40% • Wheat in India, poor year: 18% • Wheat in India, good year: 49%
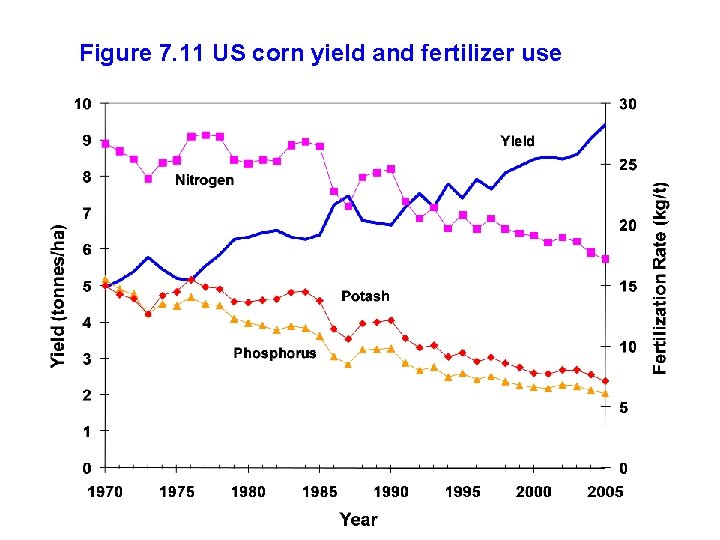
Figure 7. 11 US corn yield and fertilizer use
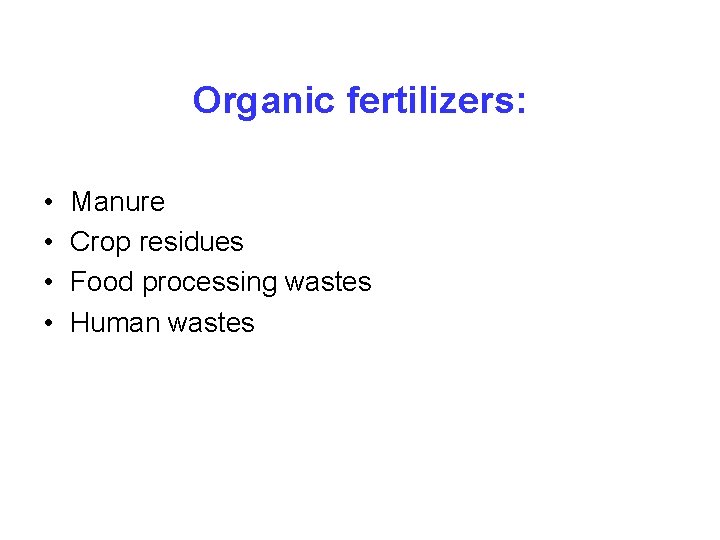
Organic fertilizers: • • Manure Crop residues Food processing wastes Human wastes
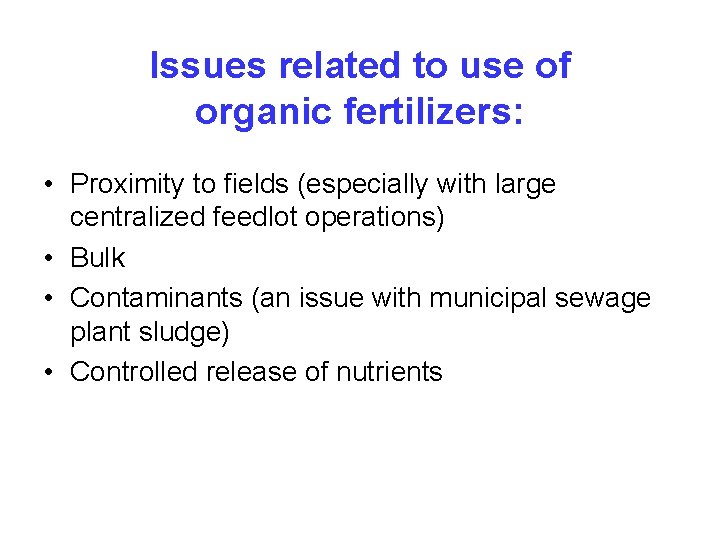
Issues related to use of organic fertilizers: • Proximity to fields (especially with large centralized feedlot operations) • Bulk • Contaminants (an issue with municipal sewage plant sludge) • Controlled release of nutrients
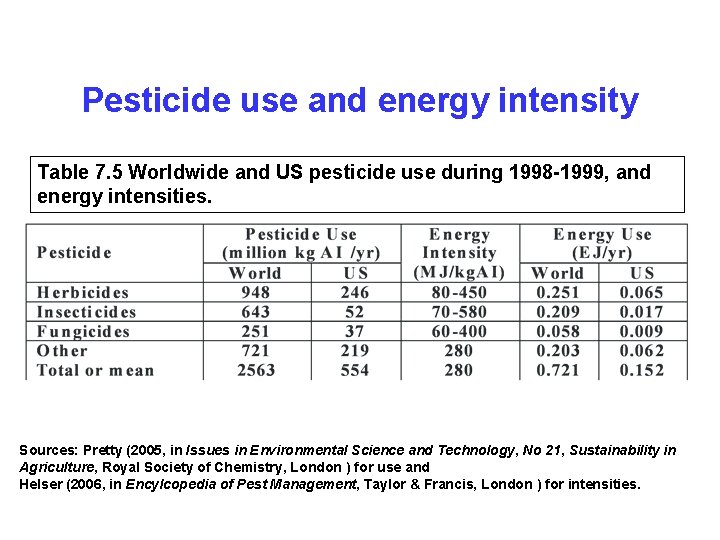
Pesticide use and energy intensity Table 7. 5 Worldwide and US pesticide use during 1998 -1999, and energy intensities. Sources: Pretty (2005, in Issues in Environmental Science and Technology, No 21, Sustainability in Agriculture, Royal Society of Chemistry, London ) for use and Helser (2006, in Encylcopedia of Pest Management, Taylor & Francis, London ) for intensities.
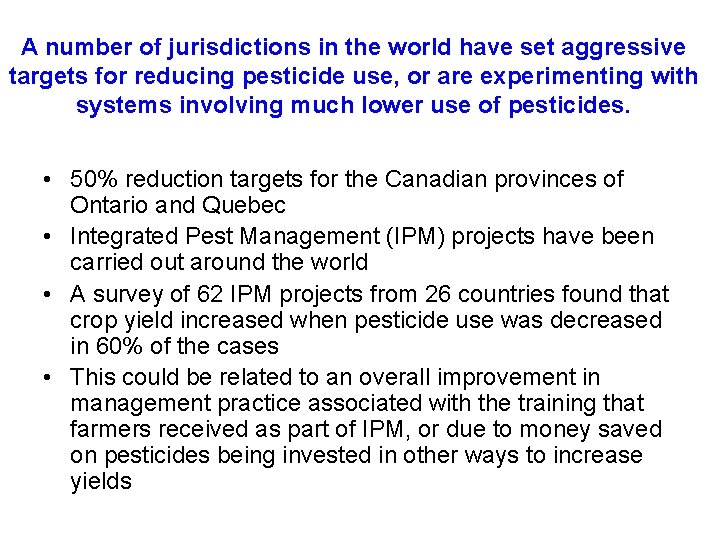
A number of jurisdictions in the world have set aggressive targets for reducing pesticide use, or are experimenting with systems involving much lower use of pesticides. • 50% reduction targets for the Canadian provinces of Ontario and Quebec • Integrated Pest Management (IPM) projects have been carried out around the world • A survey of 62 IPM projects from 26 countries found that crop yield increased when pesticide use was decreased in 60% of the cases • This could be related to an overall improvement in management practice associated with the training that farmers received as part of IPM, or due to money saved on pesticides being invested in other ways to increase yields
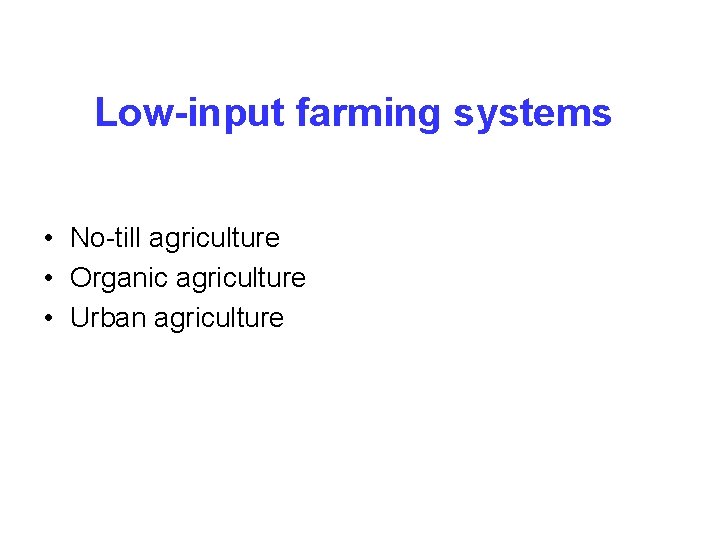
Low-input farming systems • No-till agriculture • Organic agriculture • Urban agriculture
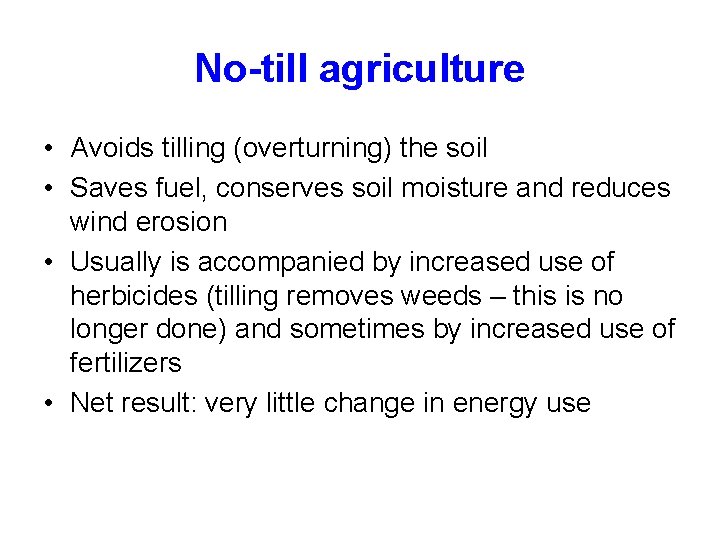
No-till agriculture • Avoids tilling (overturning) the soil • Saves fuel, conserves soil moisture and reduces wind erosion • Usually is accompanied by increased use of herbicides (tilling removes weeds – this is no longer done) and sometimes by increased use of fertilizers • Net result: very little change in energy use
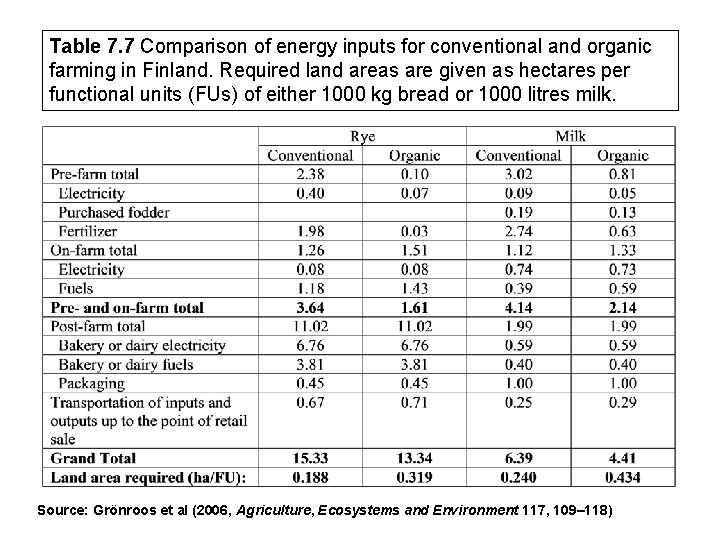
Table 7. 7 Comparison of energy inputs for conventional and organic farming in Finland. Required land areas are given as hectares per functional units (FUs) of either 1000 kg bread or 1000 litres milk. Source: Grönroos et al (2006, Agriculture, Ecosystems and Environment 117, 109– 118)
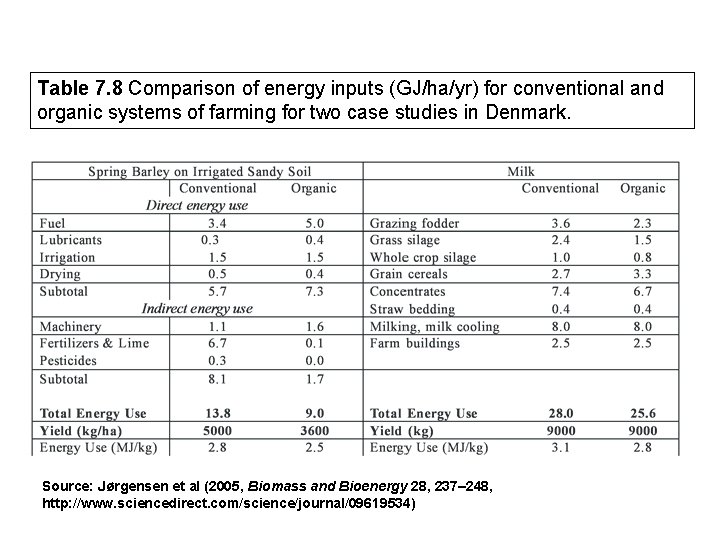
Table 7. 8 Comparison of energy inputs (GJ/ha/yr) for conventional and organic systems of farming for two case studies in Denmark. Source: Jørgensen et al (2005, Biomass and Bioenergy 28, 237– 248, http: //www. sciencedirect. com/science/journal/09619534)
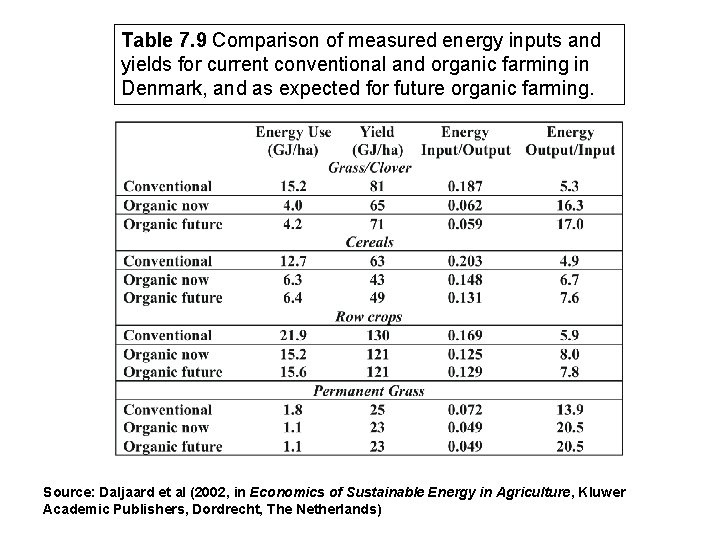
Table 7. 9 Comparison of measured energy inputs and yields for current conventional and organic farming in Denmark, and as expected for future organic farming. Source: Daljaard et al (2002, in Economics of Sustainable Energy in Agriculture, Kluwer Academic Publishers, Dordrecht, The Netherlands)
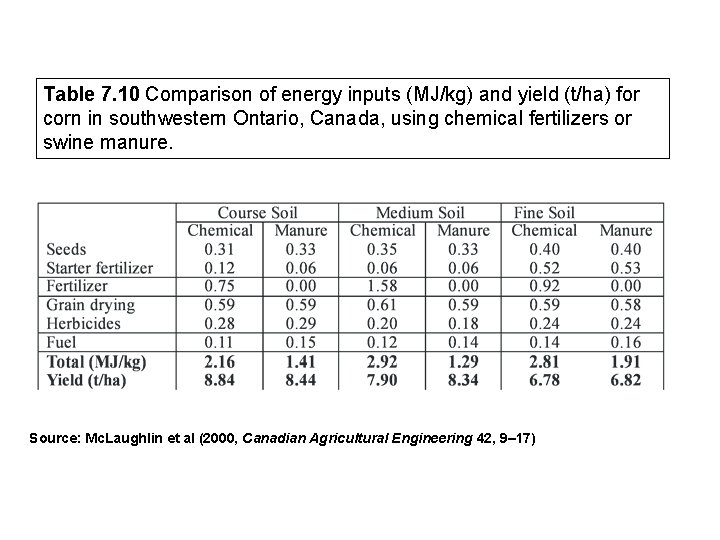
Table 7. 10 Comparison of energy inputs (MJ/kg) and yield (t/ha) for corn in southwestern Ontario, Canada, using chemical fertilizers or swine manure. Source: Mc. Laughlin et al (2000, Canadian Agricultural Engineering 42, 9– 17)
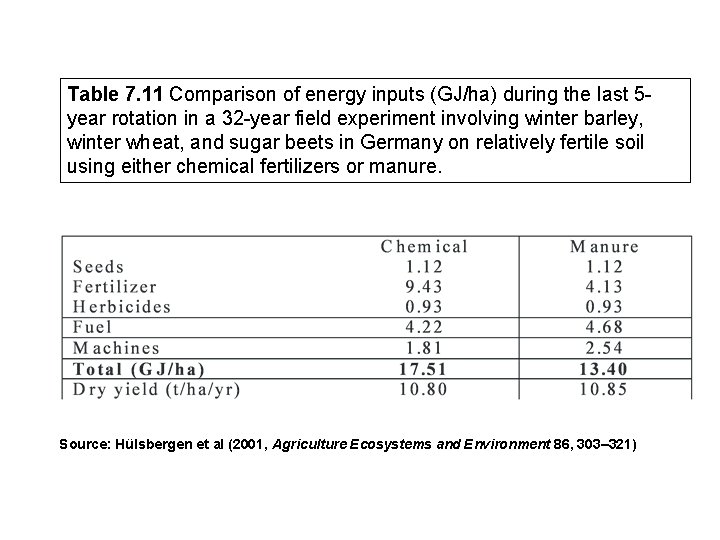
Table 7. 11 Comparison of energy inputs (GJ/ha) during the last 5 year rotation in a 32 -year field experiment involving winter barley, winter wheat, and sugar beets in Germany on relatively fertile soil using either chemical fertilizers or manure. Source: Hülsbergen et al (2001, Agriculture Ecosystems and Environment 86, 303– 321)
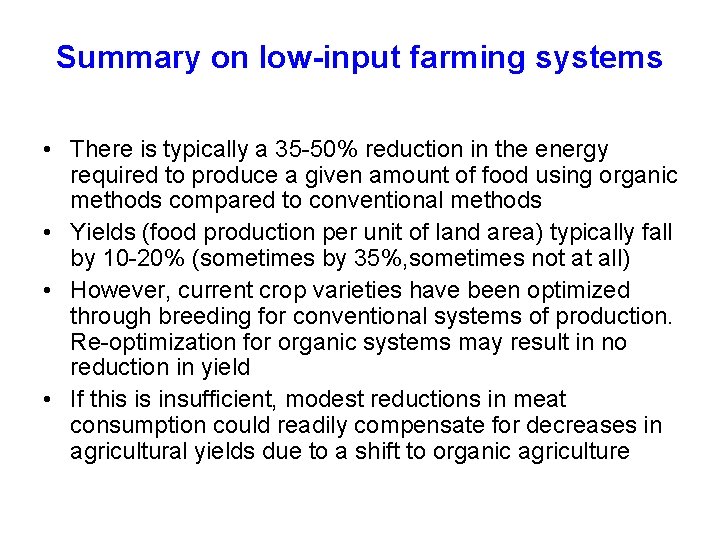
Summary on low-input farming systems • There is typically a 35 -50% reduction in the energy required to produce a given amount of food using organic methods compared to conventional methods • Yields (food production per unit of land area) typically fall by 10 -20% (sometimes by 35%, sometimes not at all) • However, current crop varieties have been optimized through breeding for conventional systems of production. Re-optimization for organic systems may result in no reduction in yield • If this is insufficient, modest reductions in meat consumption could readily compensate for decreases in agricultural yields due to a shift to organic agriculture
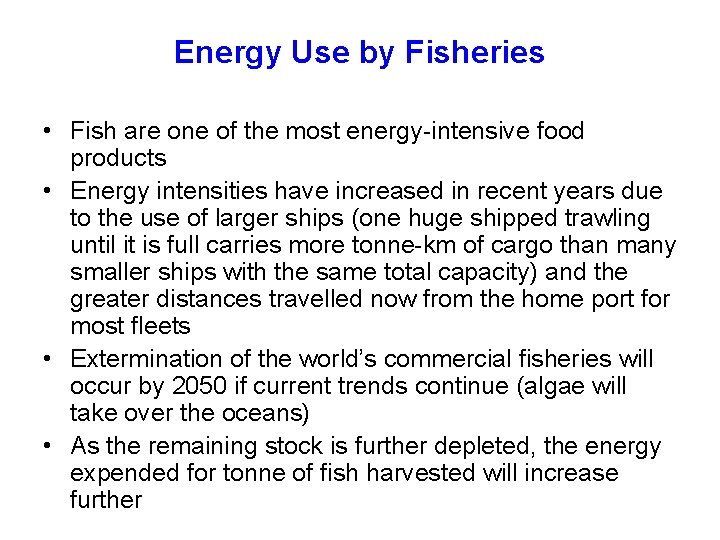
Energy Use by Fisheries • Fish are one of the most energy-intensive food products • Energy intensities have increased in recent years due to the use of larger ships (one huge shipped trawling until it is full carries more tonne-km of cargo than many smaller ships with the same total capacity) and the greater distances travelled now from the home port for most fleets • Extermination of the world’s commercial fisheries will occur by 2050 if current trends continue (algae will take over the oceans) • As the remaining stock is further depleted, the energy expended for tonne of fish harvested will increase further
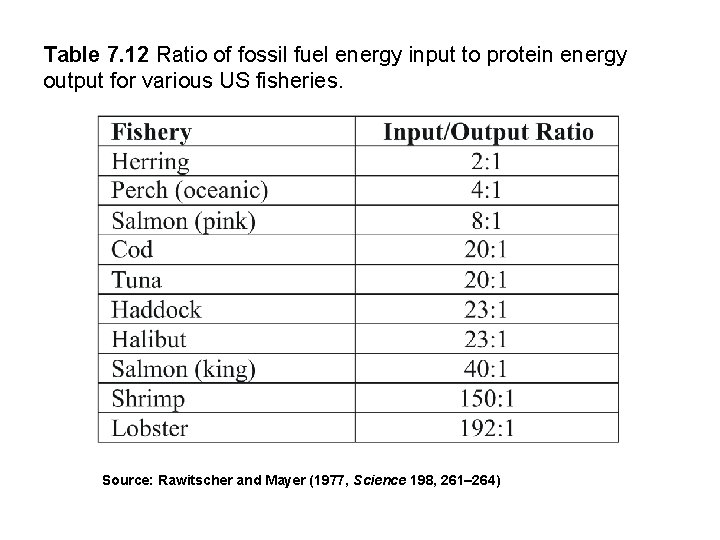
Table 7. 12 Ratio of fossil fuel energy input to protein energy output for various US fisheries. Source: Rawitscher and Mayer (1977, Science 198, 261– 264)
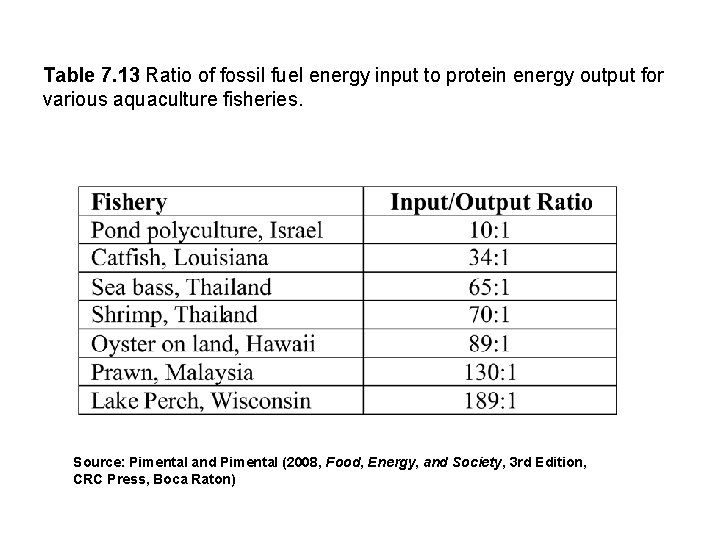
Table 7. 13 Ratio of fossil fuel energy input to protein energy output for various aquaculture fisheries. Source: Pimental and Pimental (2008, Food, Energy, and Society, 3 rd Edition, CRC Press, Boca Raton)
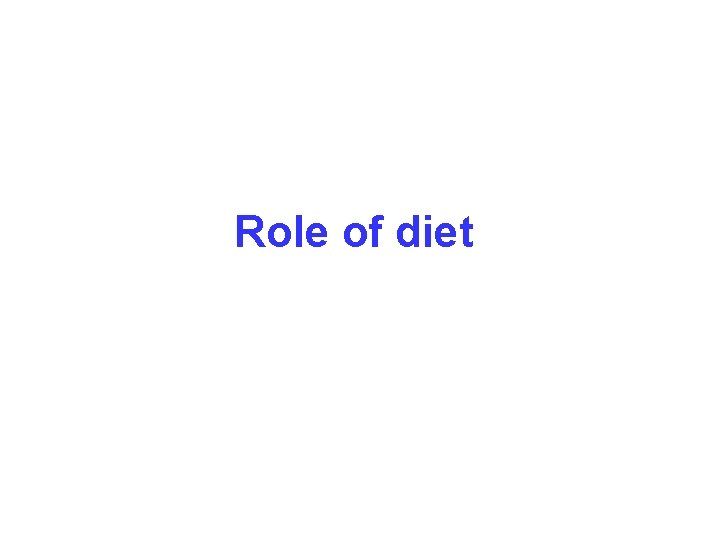
Role of diet
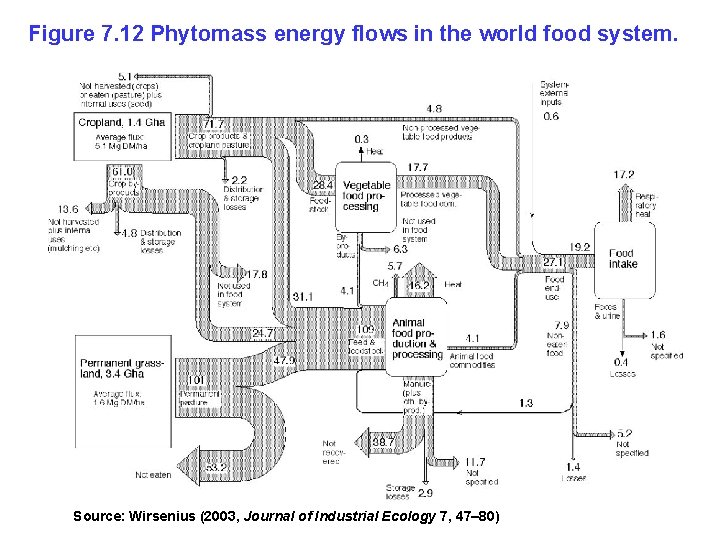
Figure 7. 12 Phytomass energy flows in the world food system. Source: Wirsenius (2003, Journal of Industrial Ecology 7, 47– 80)
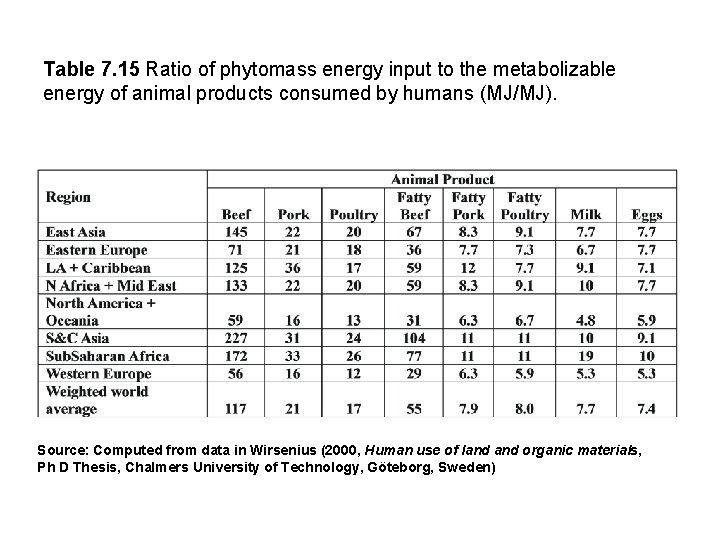
Table 7. 15 Ratio of phytomass energy input to the metabolizable energy of animal products consumed by humans (MJ/MJ). Source: Computed from data in Wirsenius (2000, Human use of land organic materials, Ph D Thesis, Chalmers University of Technology, Göteborg, Sweden)
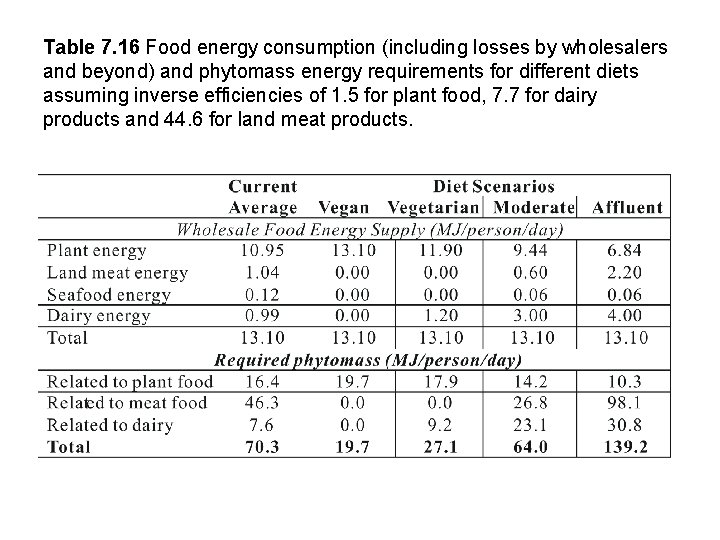
Table 7. 16 Food energy consumption (including losses by wholesalers and beyond) and phytomass energy requirements for different diets assuming inverse efficiencies of 1. 5 for plant food, 7. 7 for dairy products and 44. 6 for land meat products.
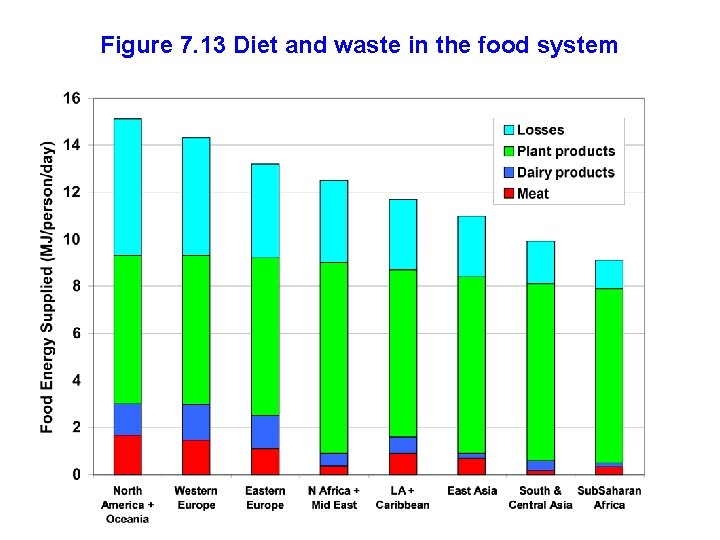
Figure 7. 13 Diet and waste in the food system
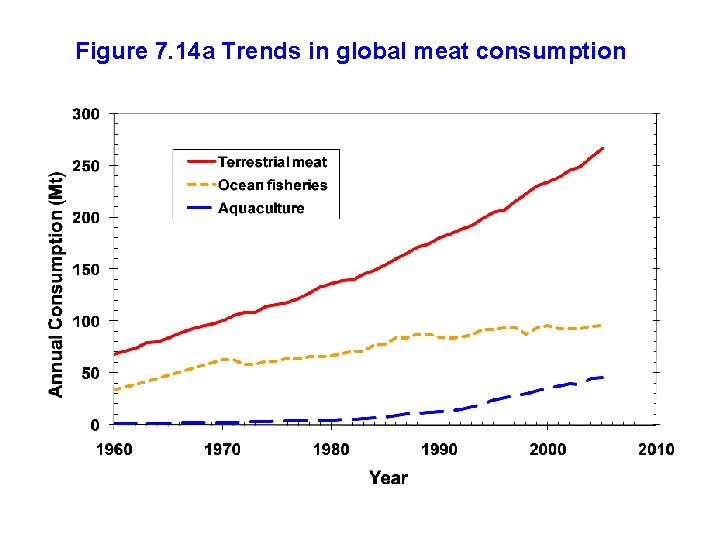
Figure 7. 14 a Trends in global meat consumption
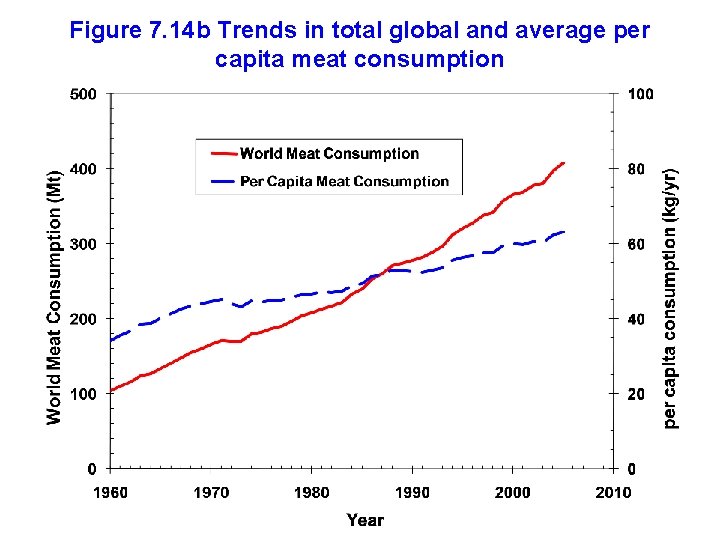
Figure 7. 14 b Trends in total global and average per capita meat consumption
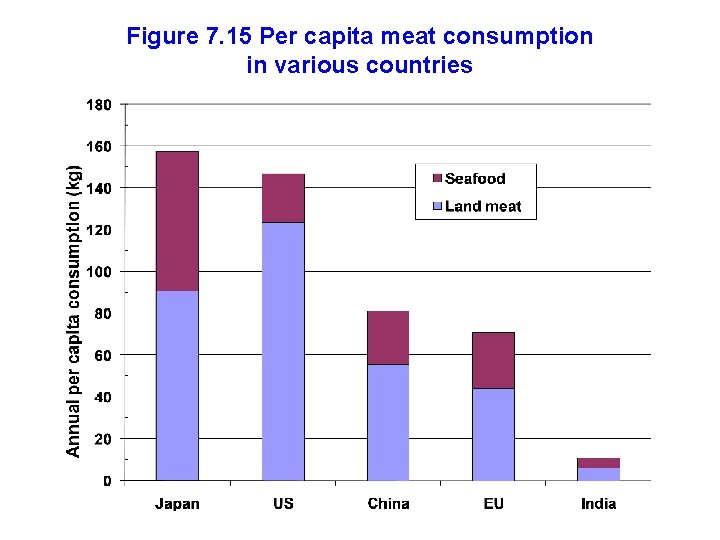
Figure 7. 15 Per capita meat consumption in various countries
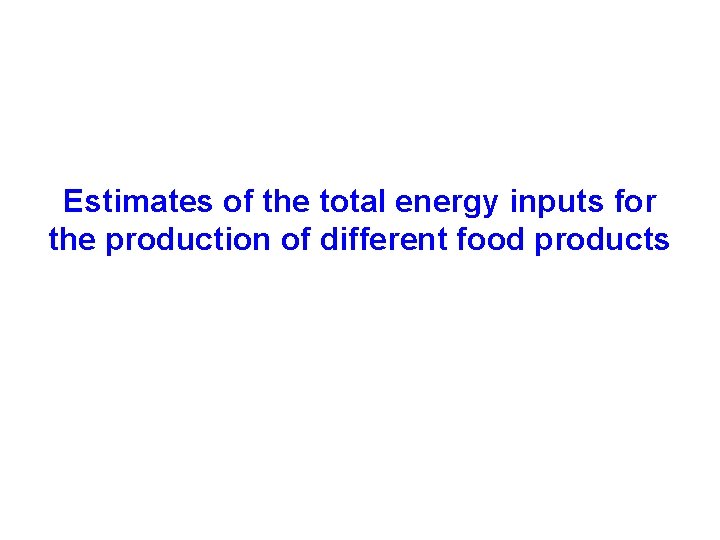
Estimates of the total energy inputs for the production of different food products
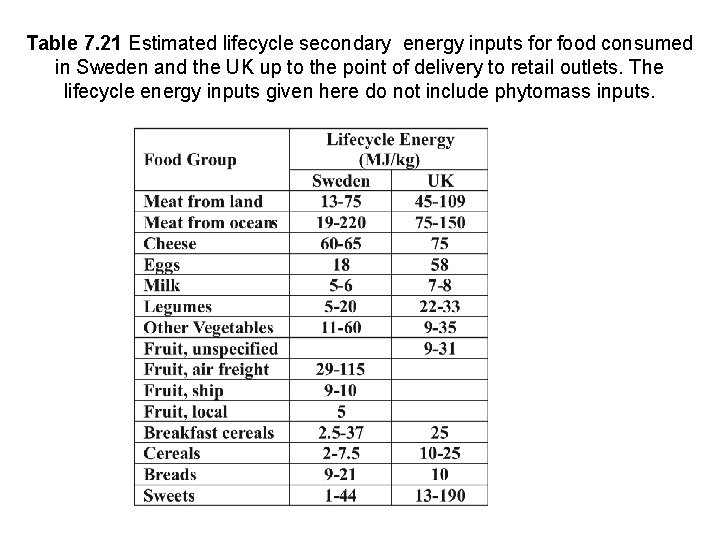
Table 7. 21 Estimated lifecycle secondary energy inputs for food consumed in Sweden and the UK up to the point of delivery to retail outlets. The lifecycle energy inputs given here do not include phytomass inputs.
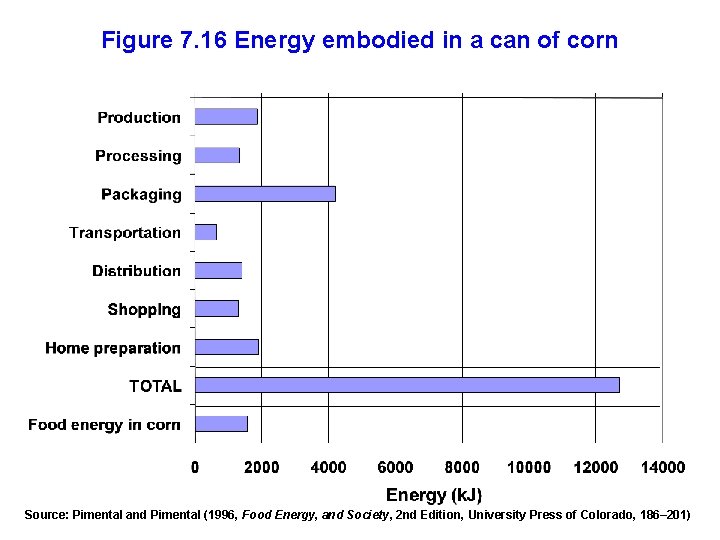
Figure 7. 16 Energy embodied in a can of corn Source: Pimental and Pimental (1996, Food Energy, and Society, 2 nd Edition, University Press of Colorado, 186– 201)
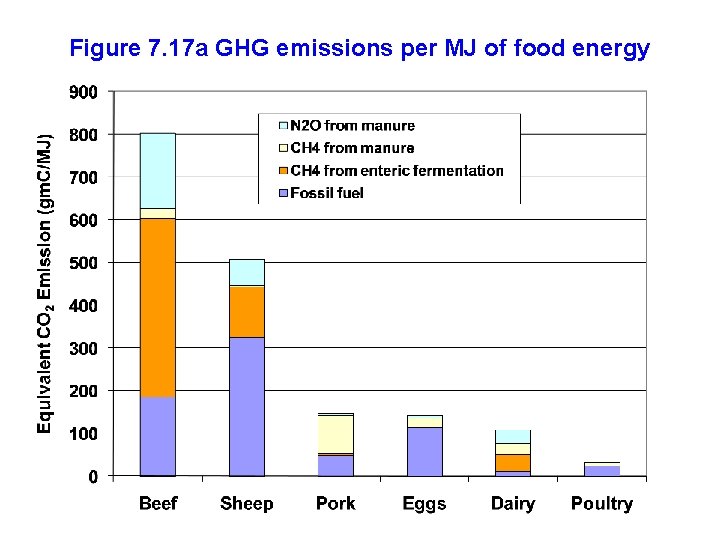
Figure 7. 17 a GHG emissions per MJ of food energy
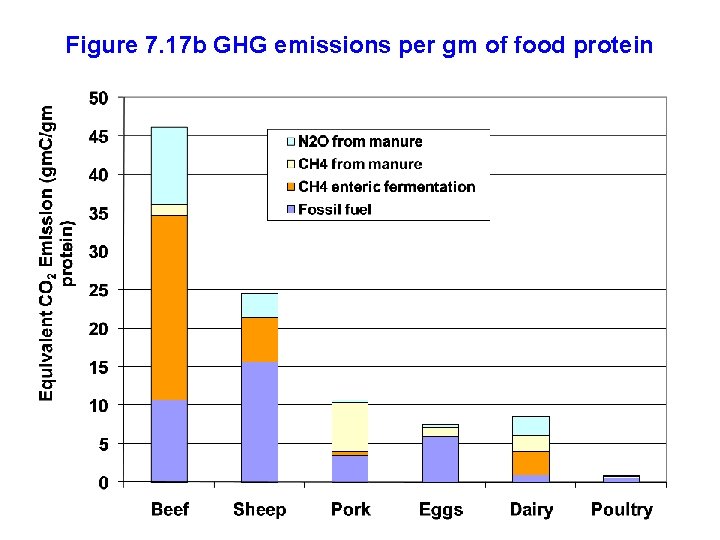
Figure 7. 17 b GHG emissions per gm of food protein
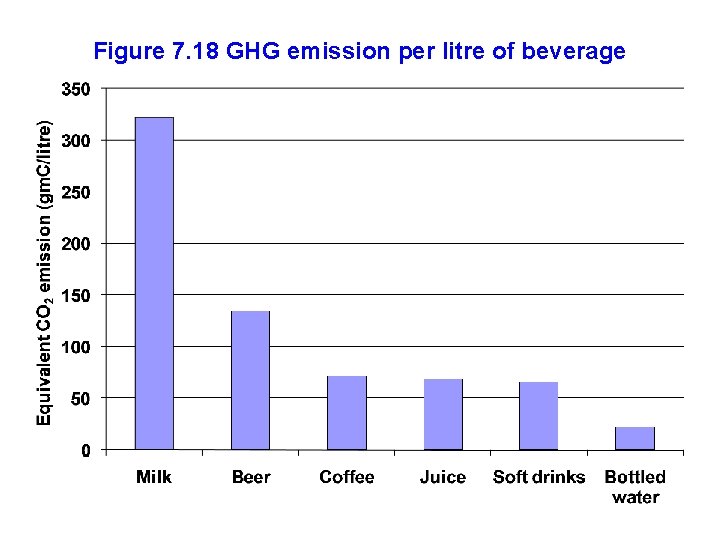
Figure 7. 18 GHG emission per litre of beverage
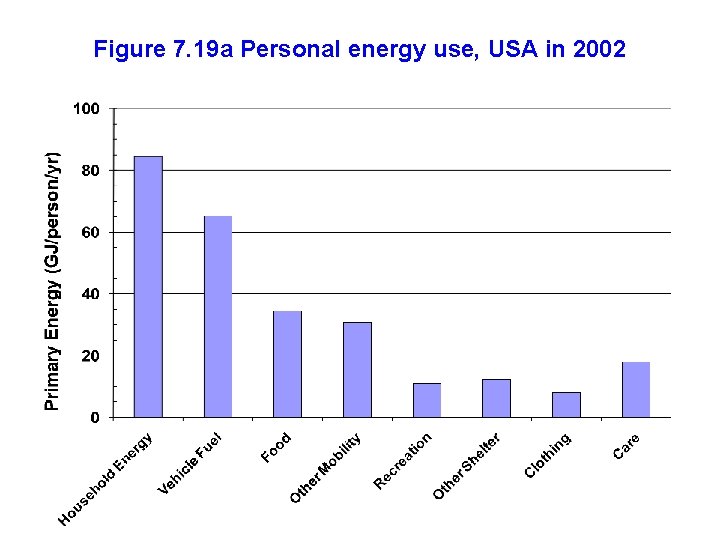
Figure 7. 19 a Personal energy use, USA in 2002
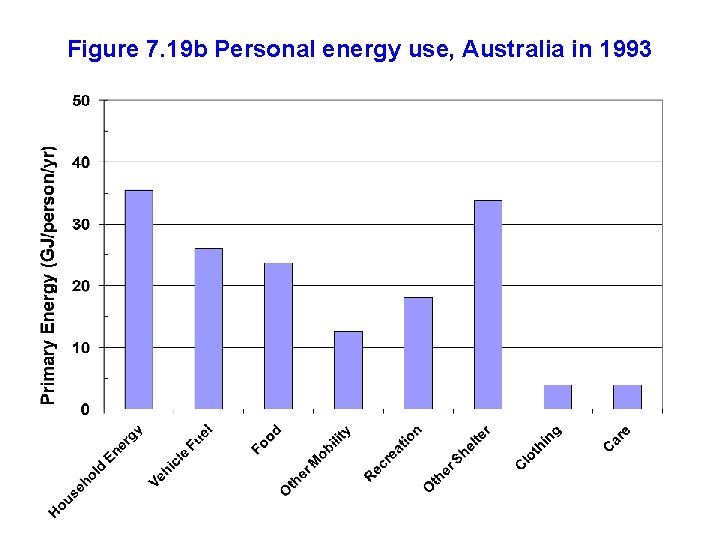
Figure 7. 19 b Personal energy use, Australia in 1993
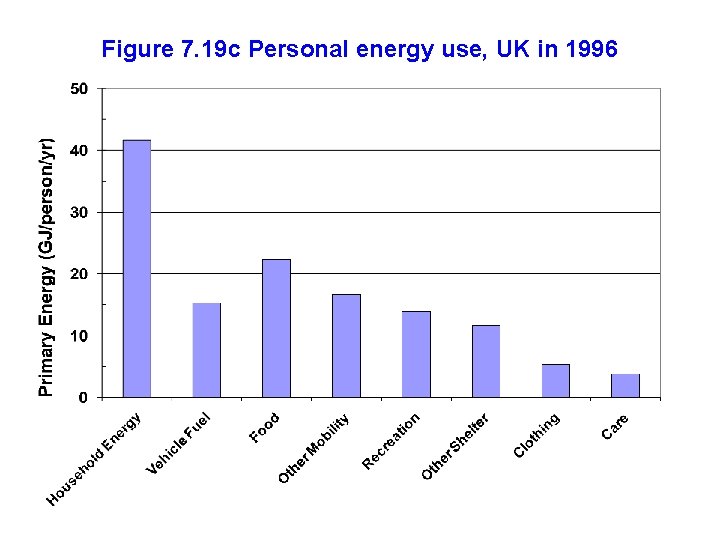
Figure 7. 19 c Personal energy use, UK in 1996
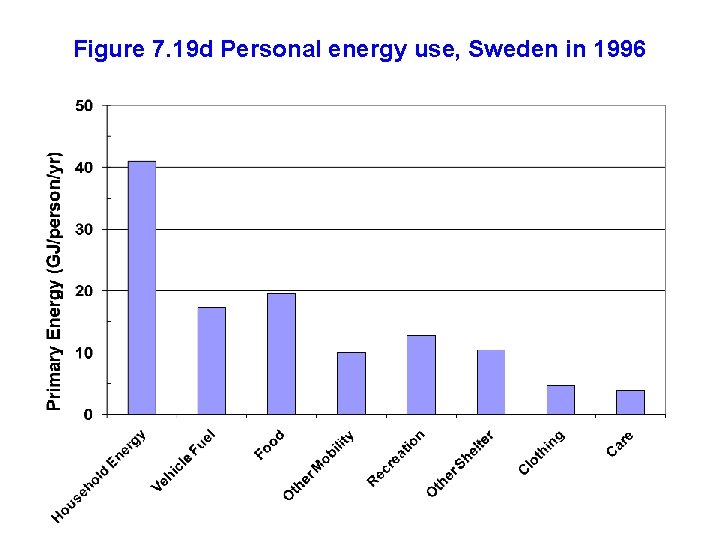
Figure 7. 19 d Personal energy use, Sweden in 1996
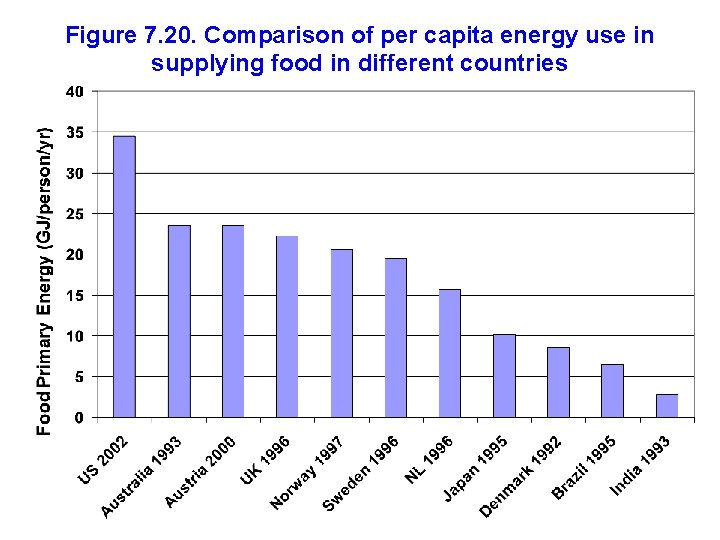
Figure 7. 20. Comparison of per capita energy use in supplying food in different countries
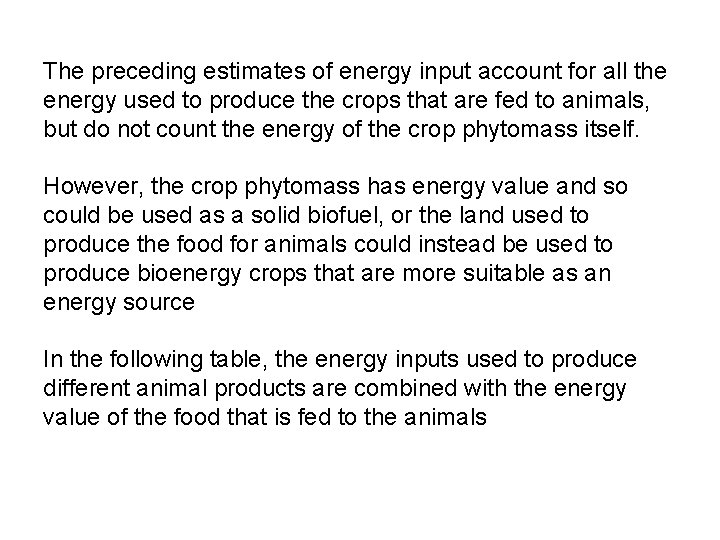
The preceding estimates of energy input account for all the energy used to produce the crops that are fed to animals, but do not count the energy of the crop phytomass itself. However, the crop phytomass has energy value and so could be used as a solid biofuel, or the land used to produce the food for animals could instead be used to produce bioenergy crops that are more suitable as an energy source In the following table, the energy inputs used to produce different animal products are combined with the energy value of the food that is fed to the animals
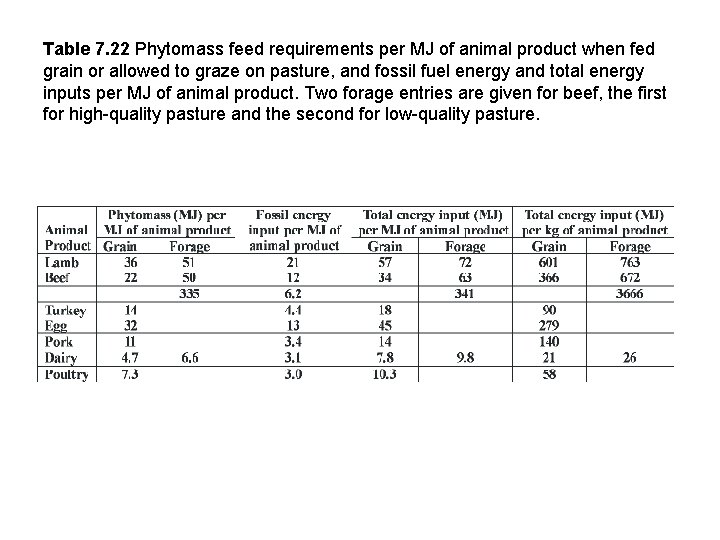
Table 7. 22 Phytomass feed requirements per MJ of animal product when fed grain or allowed to graze on pasture, and fossil fuel energy and total energy inputs per MJ of animal product. Two forage entries are given for beef, the first for high-quality pasture and the second for low-quality pasture.
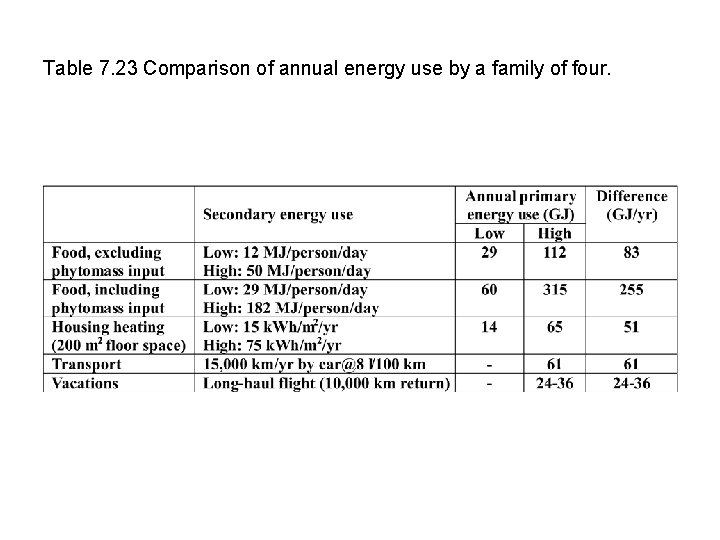
Table 7. 23 Comparison of annual energy use by a family of four.
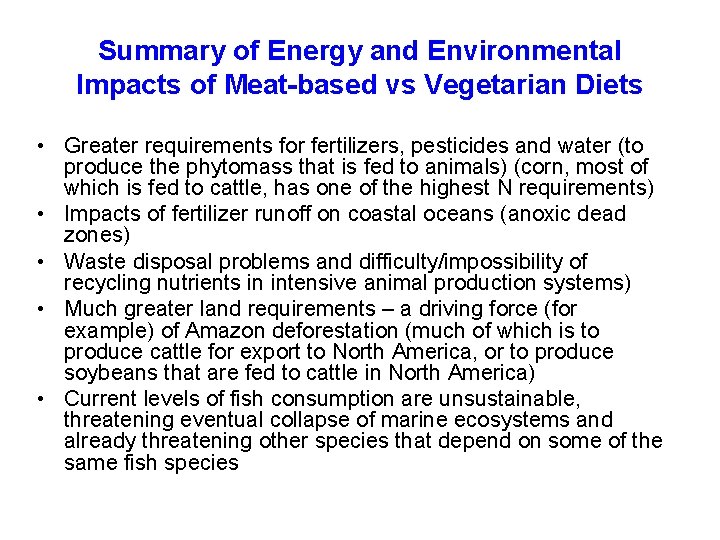
Summary of Energy and Environmental Impacts of Meat-based vs Vegetarian Diets • Greater requirements for fertilizers, pesticides and water (to produce the phytomass that is fed to animals) (corn, most of which is fed to cattle, has one of the highest N requirements) • Impacts of fertilizer runoff on coastal oceans (anoxic dead zones) • Waste disposal problems and difficulty/impossibility of recycling nutrients in intensive animal production systems) • Much greater land requirements – a driving force (for example) of Amazon deforestation (much of which is to produce cattle for export to North America, or to produce soybeans that are fed to cattle in North America) • Current levels of fish consumption are unsustainable, threatening eventual collapse of marine ecosystems and already threatening other species that depend on some of the same fish species
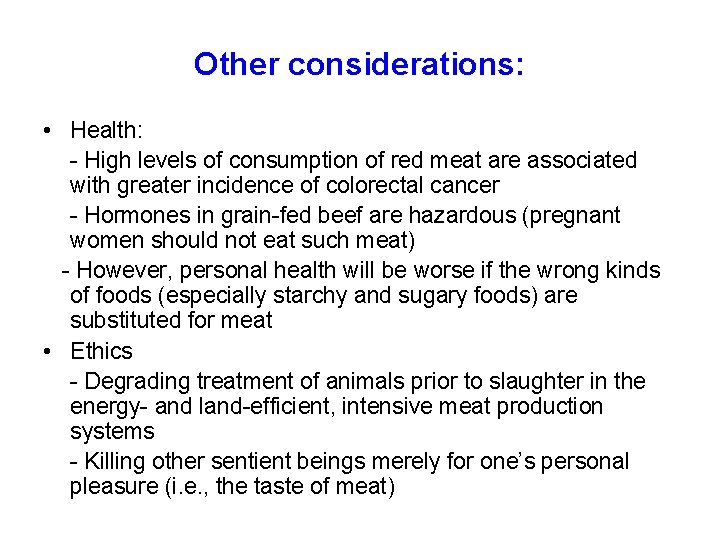
Other considerations: • Health: - High levels of consumption of red meat are associated with greater incidence of colorectal cancer - Hormones in grain-fed beef are hazardous (pregnant women should not eat such meat) - However, personal health will be worse if the wrong kinds of foods (especially starchy and sugary foods) are substituted for meat • Ethics - Degrading treatment of animals prior to slaughter in the energy- and land-efficient, intensive meat production systems - Killing other sentient beings merely for one’s personal pleasure (i. e. , the taste of meat)
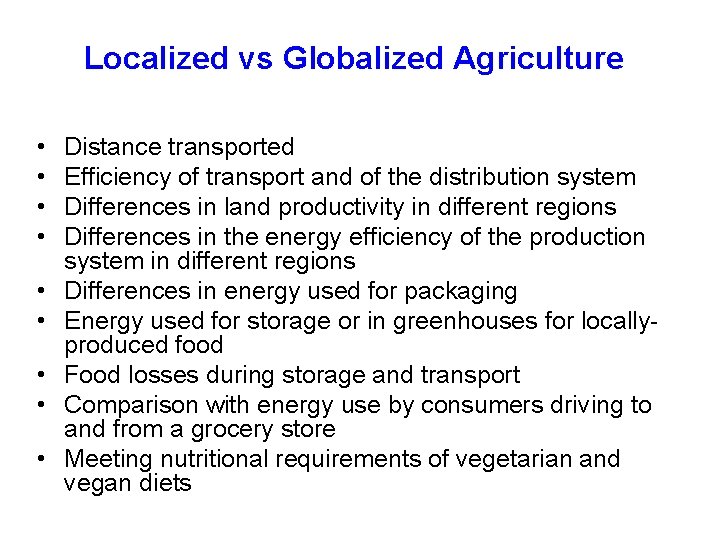
Localized vs Globalized Agriculture • • • Distance transported Efficiency of transport and of the distribution system Differences in land productivity in different regions Differences in the energy efficiency of the production system in different regions Differences in energy used for packaging Energy used for storage or in greenhouses for locallyproduced food Food losses during storage and transport Comparison with energy use by consumers driving to and from a grocery store Meeting nutritional requirements of vegetarian and vegan diets
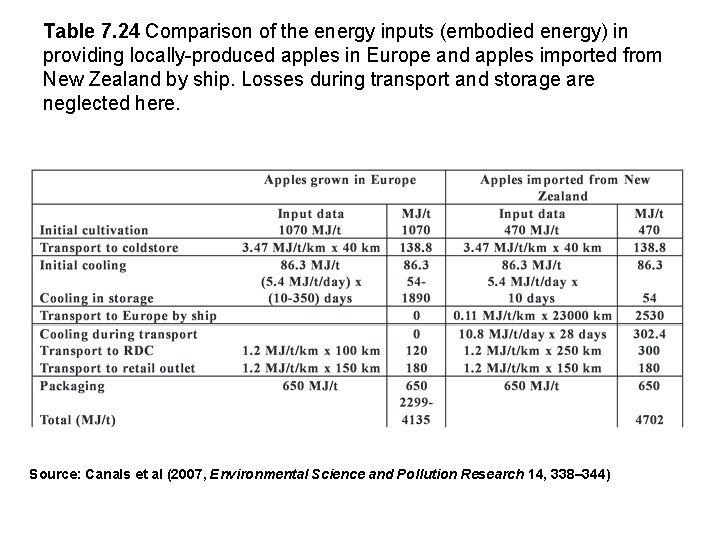
Table 7. 24 Comparison of the energy inputs (embodied energy) in providing locally-produced apples in Europe and apples imported from New Zealand by ship. Losses during transport and storage are neglected here. Source: Canals et al (2007, Environmental Science and Pollution Research 14, 338– 344)
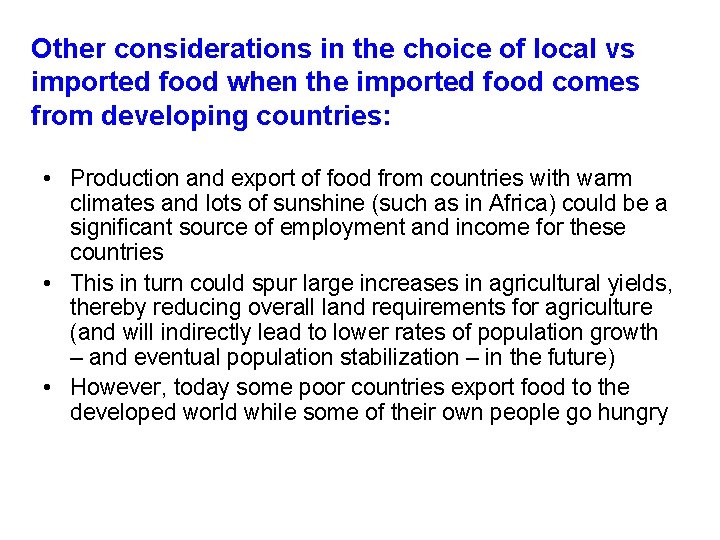
Other considerations in the choice of local vs imported food when the imported food comes from developing countries: • Production and export of food from countries with warm climates and lots of sunshine (such as in Africa) could be a significant source of employment and income for these countries • This in turn could spur large increases in agricultural yields, thereby reducing overall land requirements for agriculture (and will indirectly lead to lower rates of population growth – and eventual population stabilization – in the future) • However, today some poor countries export food to the developed world while some of their own people go hungry
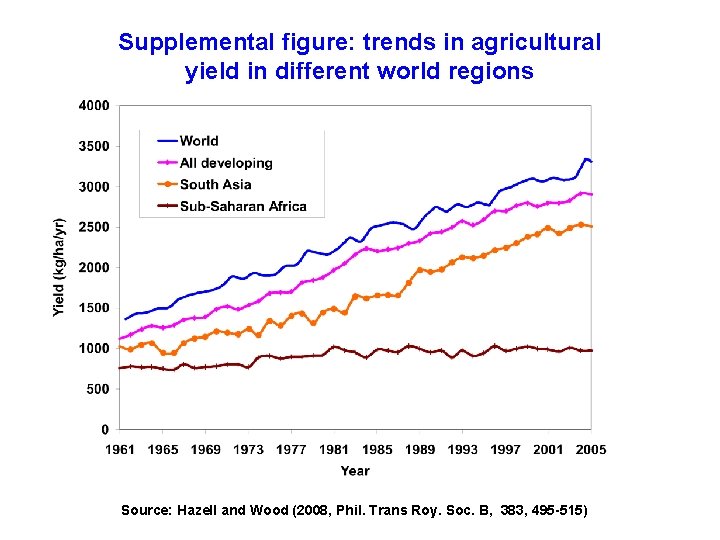
Supplemental figure: trends in agricultural yield in different world regions Source: Hazell and Wood (2008, Phil. Trans Roy. Soc. B, 383, 495 -515)
Energy energy transfer and general energy analysis
Energy energy transfer and general energy analysis
Stroke volume units
Edv and preload
Volume of solute divided by volume of solution * 100
Lung capacity
Volume kerucut = .....x volume tabung *
Large volume parenterals examples
Hát kết hợp bộ gõ cơ thể
Slidetodoc
Bổ thể
Tỉ lệ cơ thể trẻ em
Voi kéo gỗ như thế nào
Tư thế worms-breton
Chúa yêu trần thế
Môn thể thao bắt đầu bằng chữ đua
Thế nào là hệ số cao nhất
Các châu lục và đại dương trên thế giới
Công của trọng lực
Trời xanh đây là của chúng ta thể thơ
Mật thư tọa độ 5x5
101012 bằng
độ dài liên kết
Các châu lục và đại dương trên thế giới
Thể thơ truyền thống
Quá trình desamine hóa có thể tạo ra
Một số thể thơ truyền thống
Cái miệng nó xinh thế chỉ nói điều hay thôi
Vẽ hình chiếu vuông góc của vật thể sau
Thế nào là sự mỏi cơ
đặc điểm cơ thể của người tối cổ
Giọng cùng tên là
Vẽ hình chiếu đứng bằng cạnh của vật thể
Tia chieu sa te
Thẻ vin
đại từ thay thế
điện thế nghỉ
Tư thế ngồi viết
Diễn thế sinh thái là
Dạng đột biến một nhiễm là
Số nguyên tố là số gì
Tư thế ngồi viết
Lời thề hippocrates
Thiếu nhi thế giới liên hoan
ưu thế lai là gì
Sự nuôi và dạy con của hổ
Sự nuôi và dạy con của hổ
Sơ đồ cơ thể người
Từ ngữ thể hiện lòng nhân hậu
Thế nào là mạng điện lắp đặt kiểu nổi
New york pennsylvania new jersey delaware
Fresh oil, new wine scripture
Articles of confederation strengths
Kotler and keller
New classical macroeconomics
Chapter 16 toward a new heaven and a new earth
Leanne keene french ambassador arrives from paris
New classical and new keynesian macroeconomics
Cefr companion
Vr input and output devices
The merchant of venice appearance vs reality
Zoos connect us to the natural world
What is sarcasm
Augmented reality: principles and practice
Vision focuses on the current reality and maintaining it
Generally restful like the horizon where the sky meets land
Shadow ot
Pros and cons of virtual reality in healthcare
Np complete
Hilda tresz
Examples of inverted commas
Marquee cinema