Chemistry 20 Ch 4 Gases Solids Liquids Gases
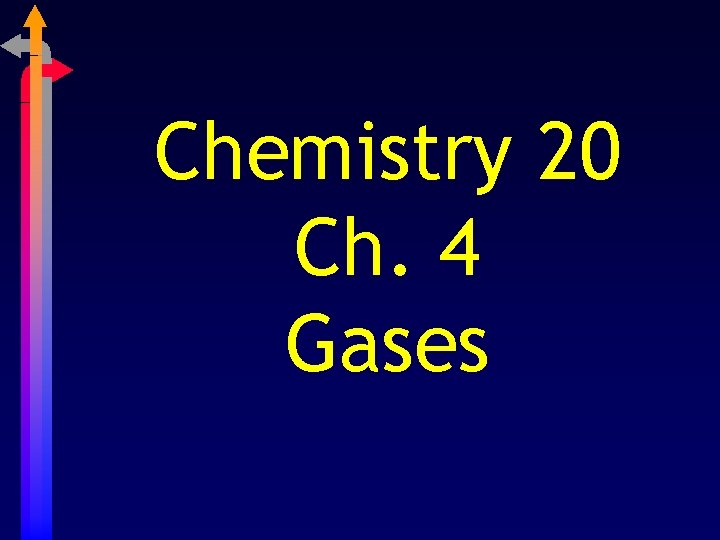
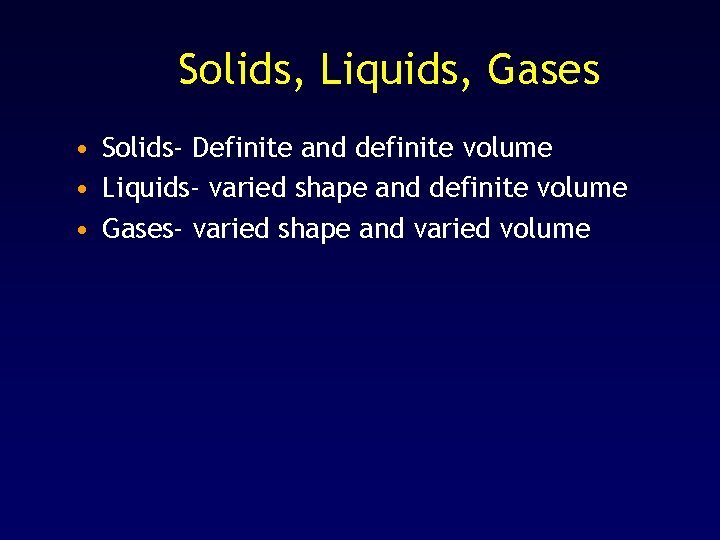
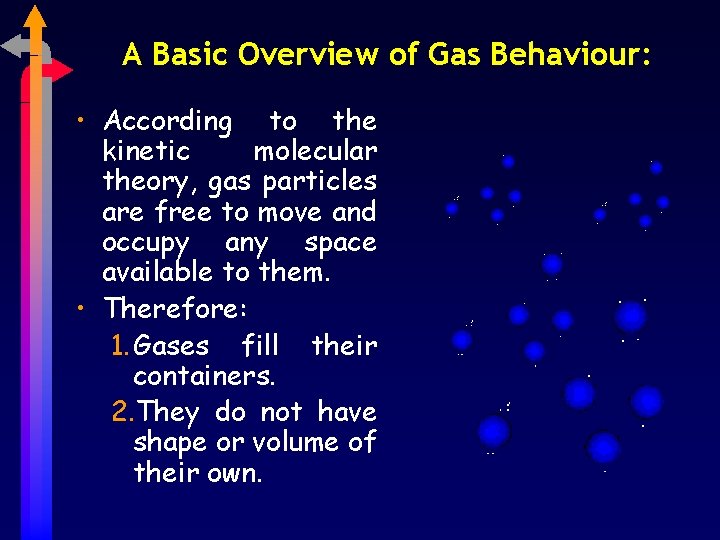
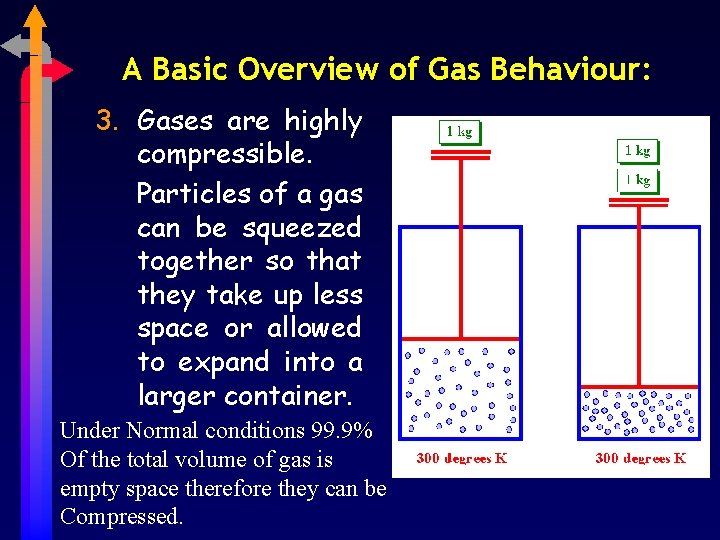
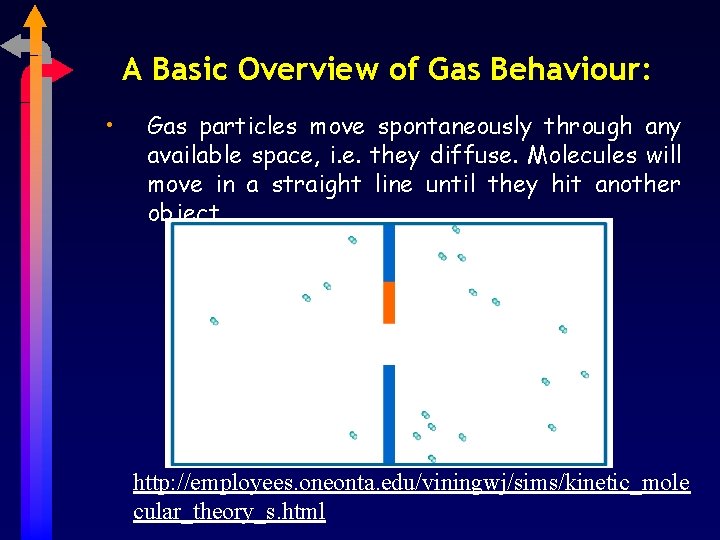
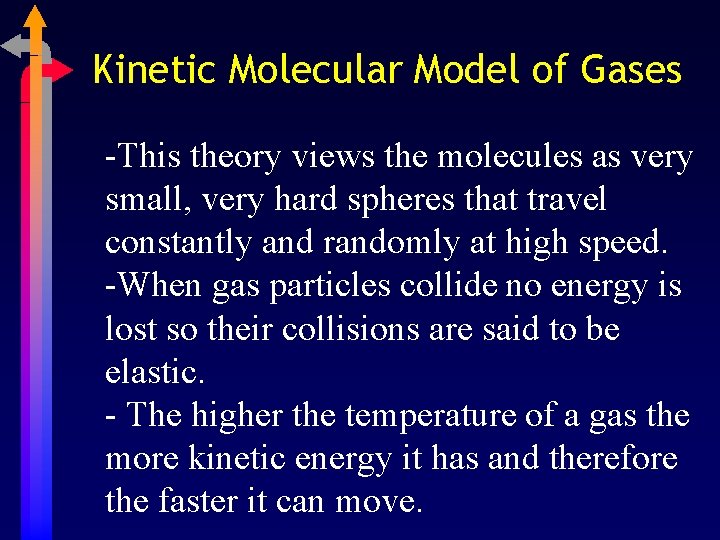
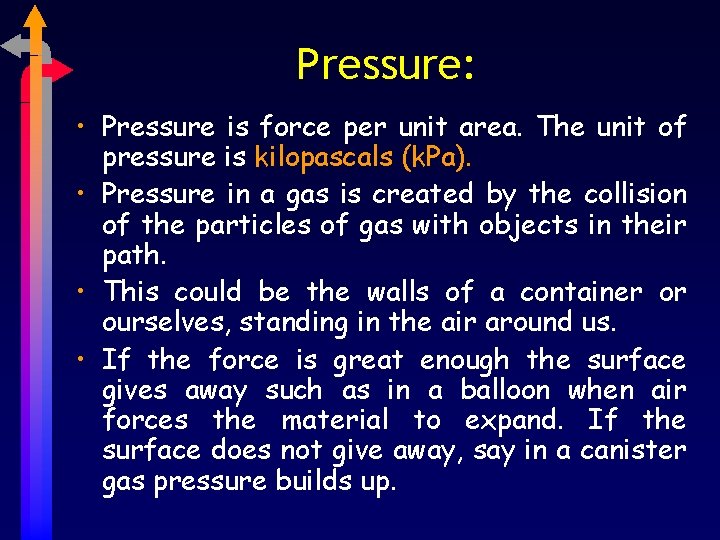
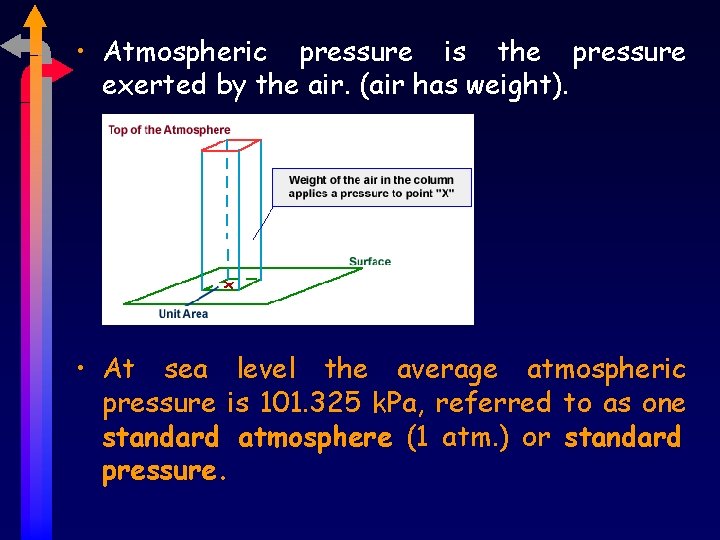
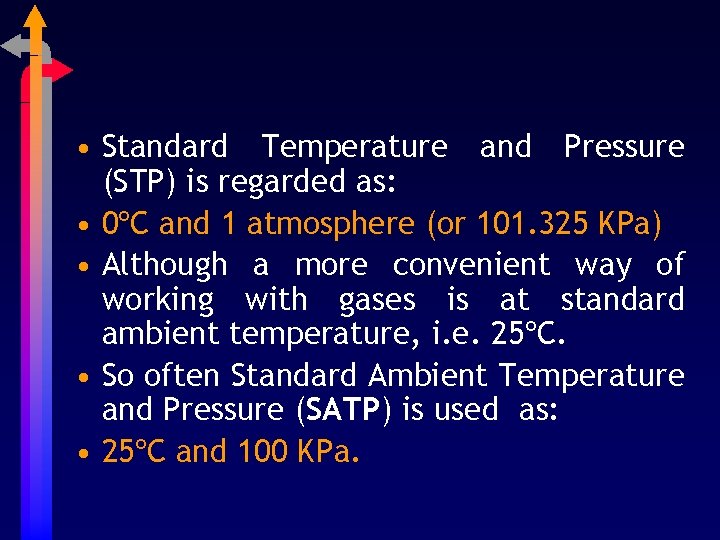
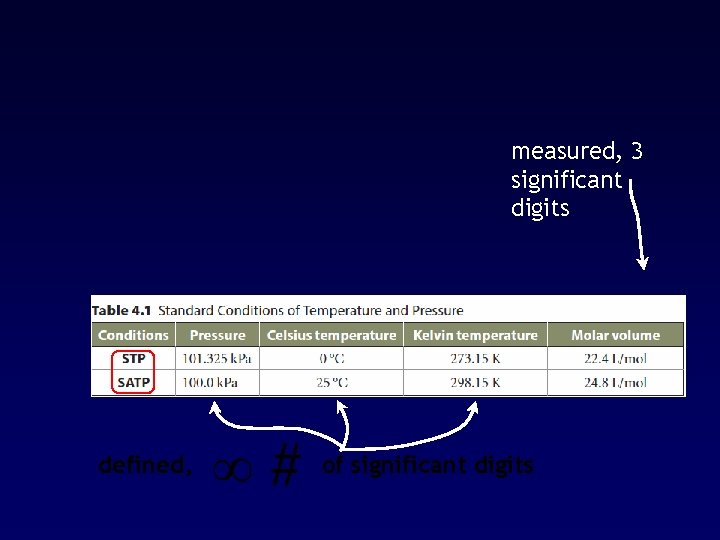
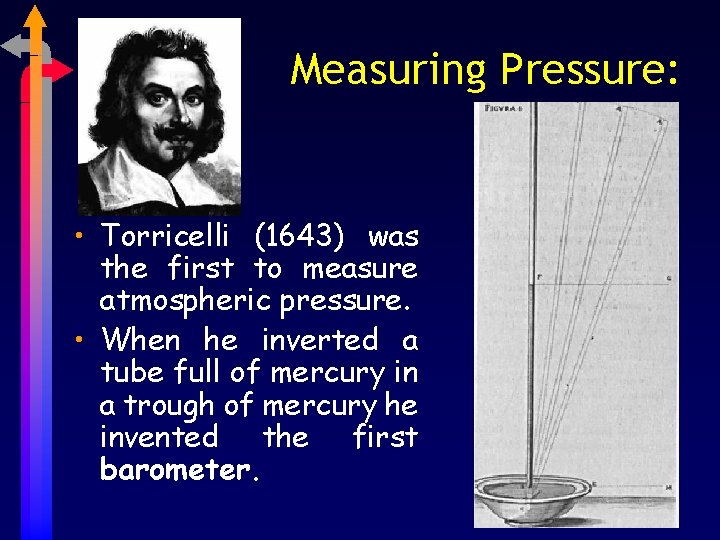
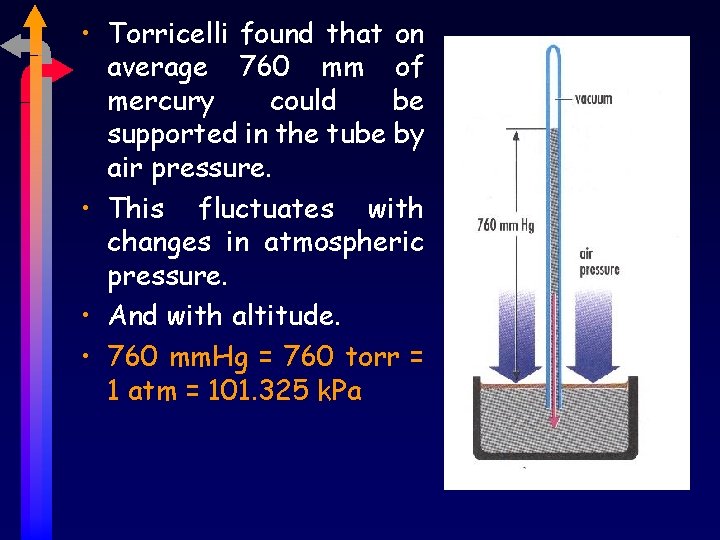
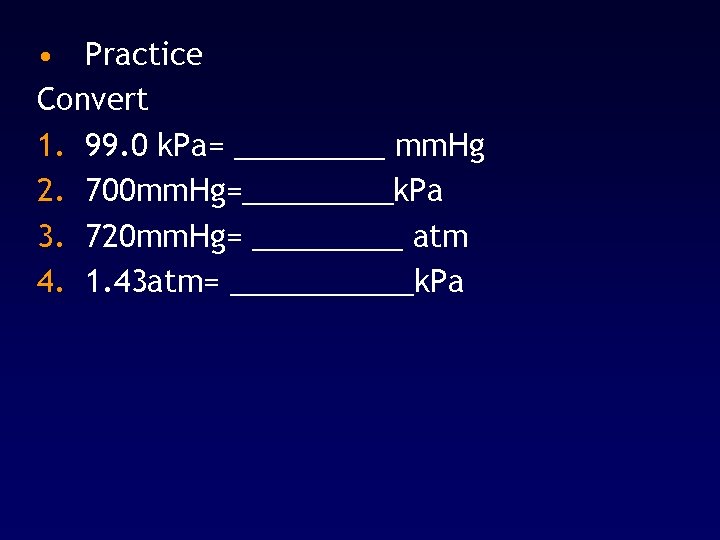
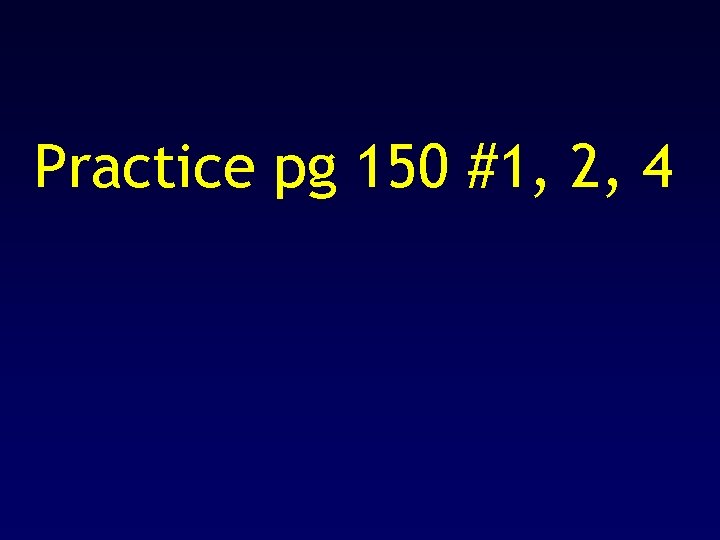
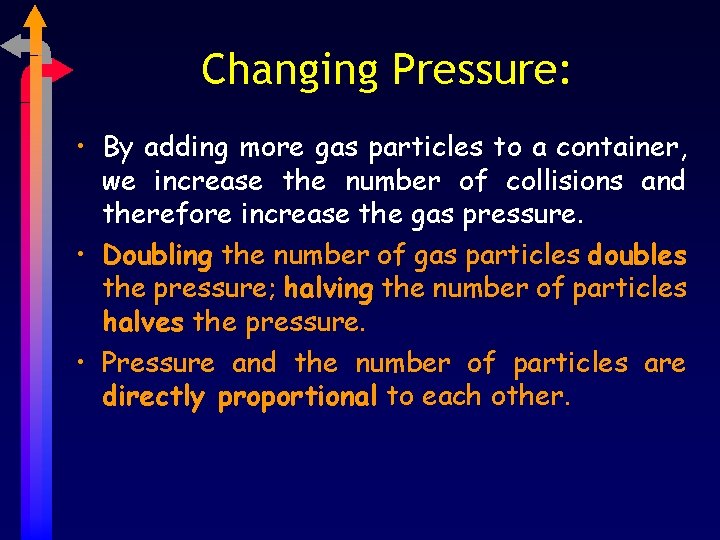
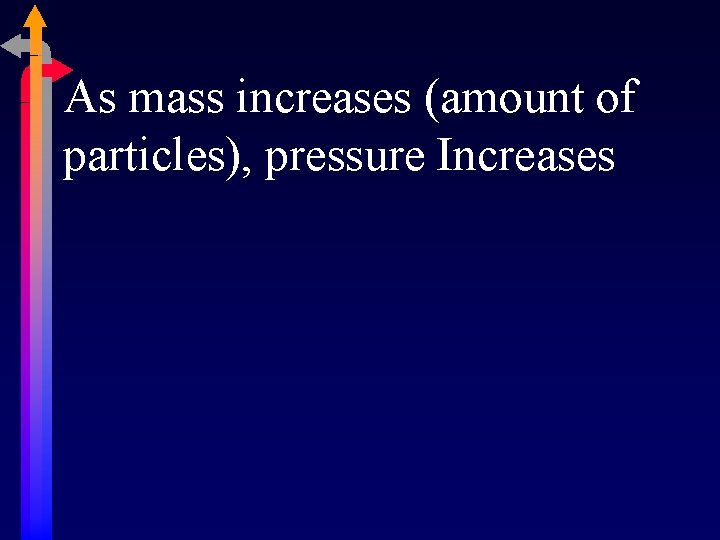
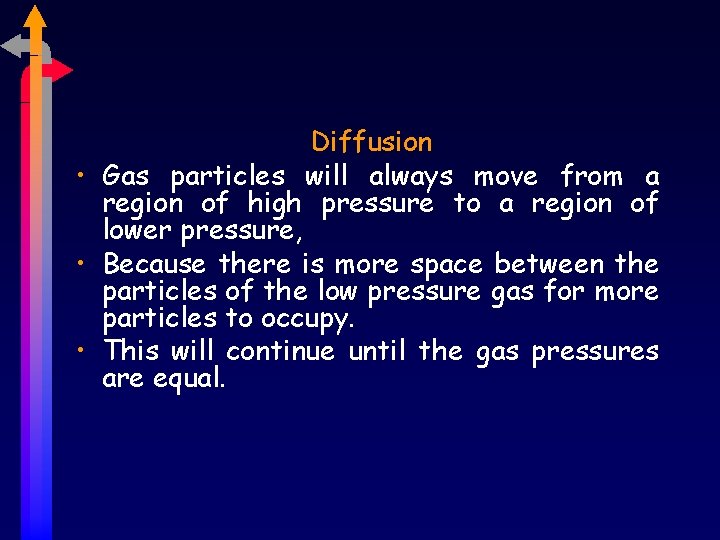
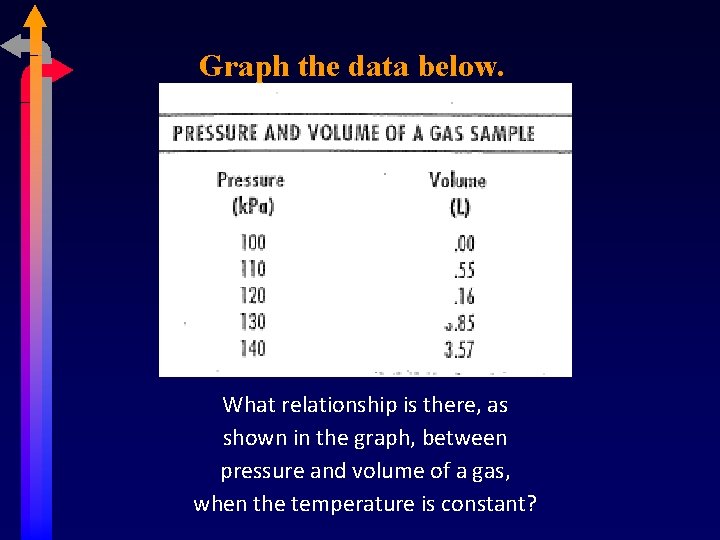
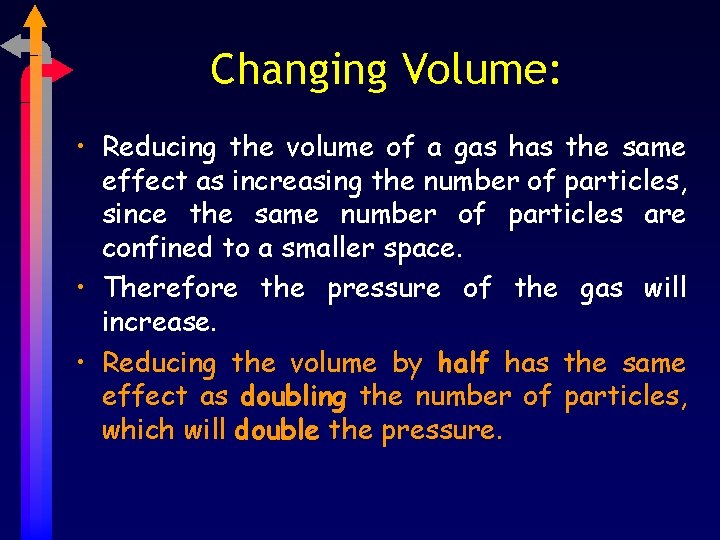
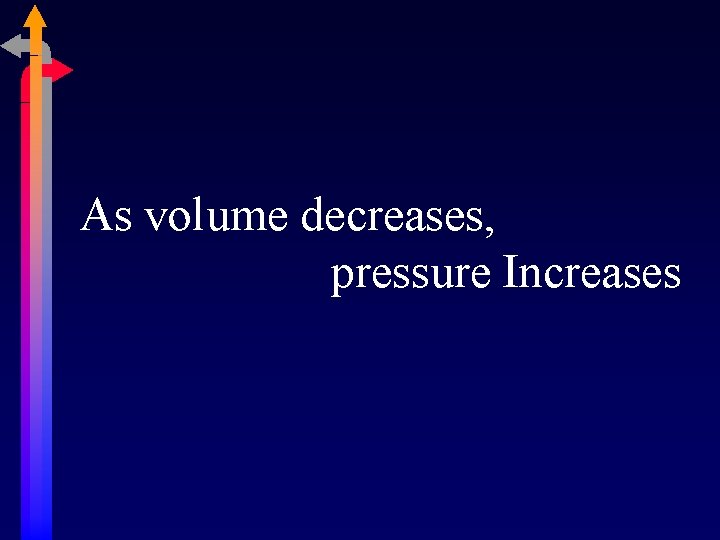
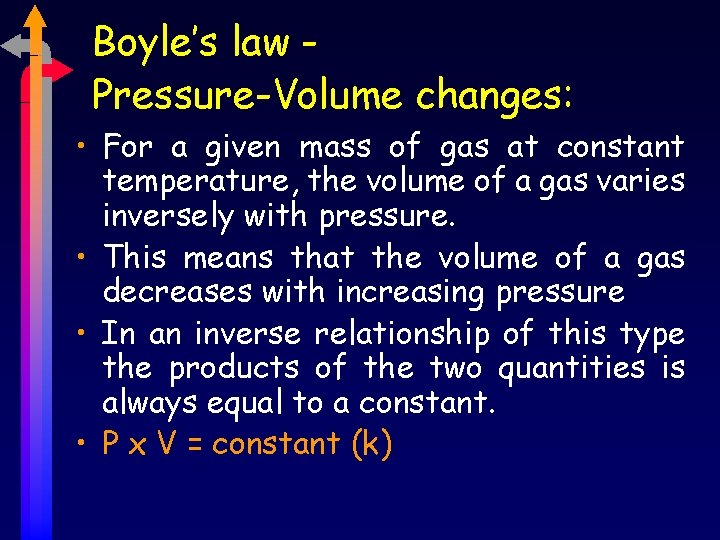
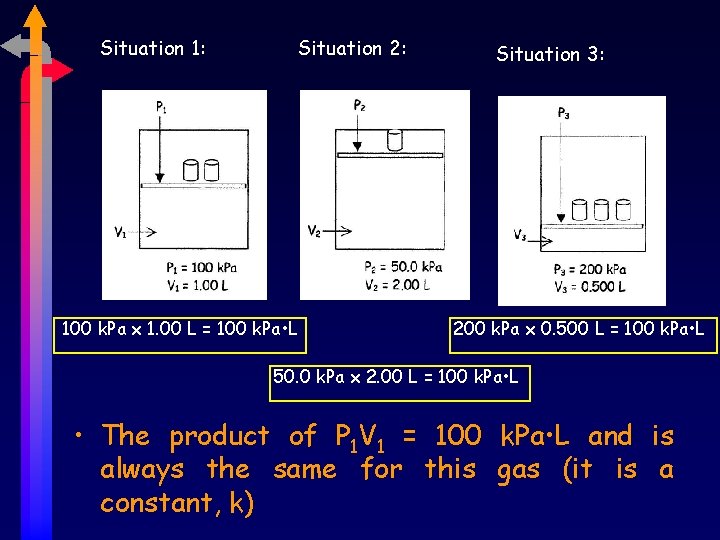
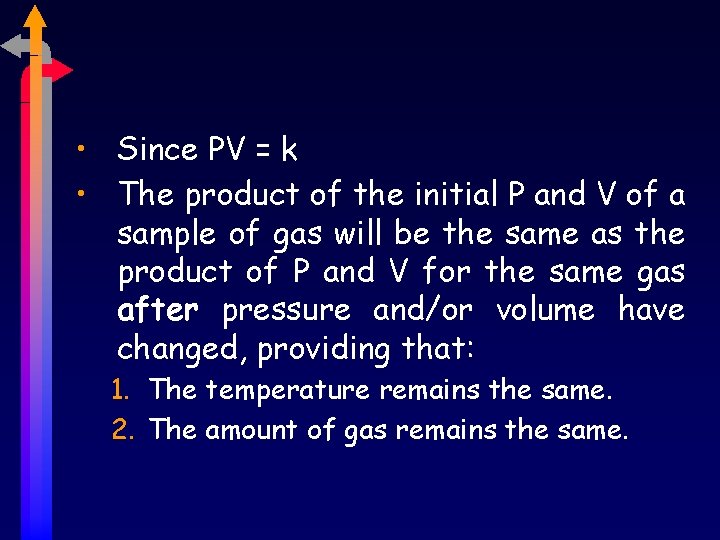
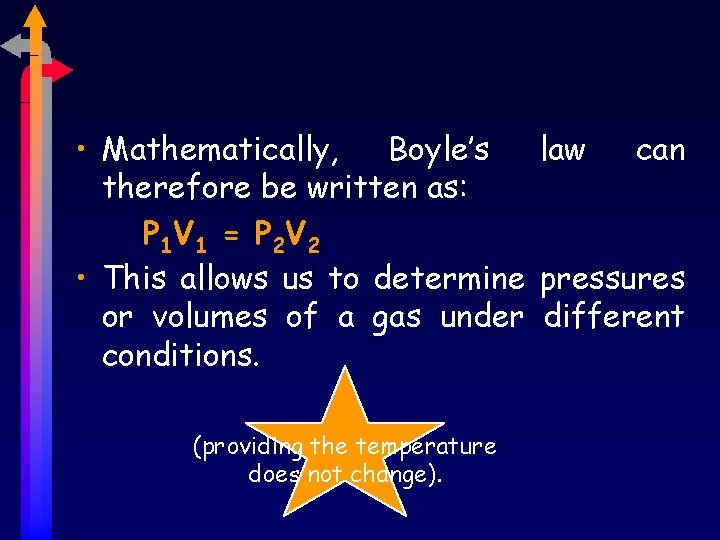
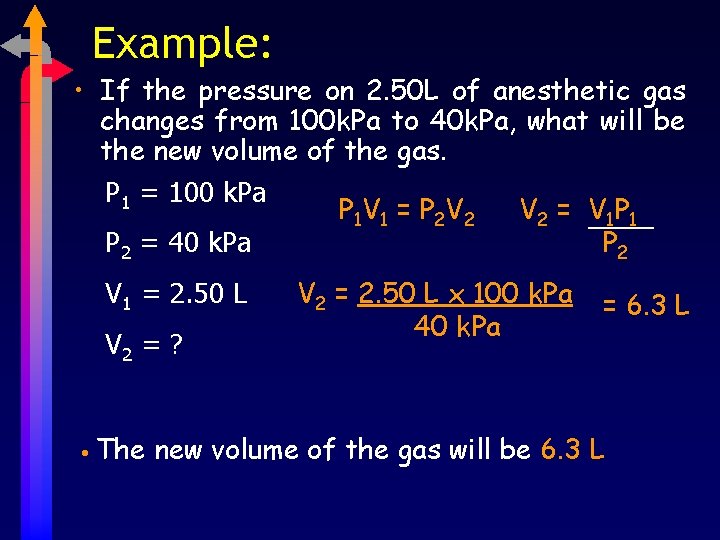
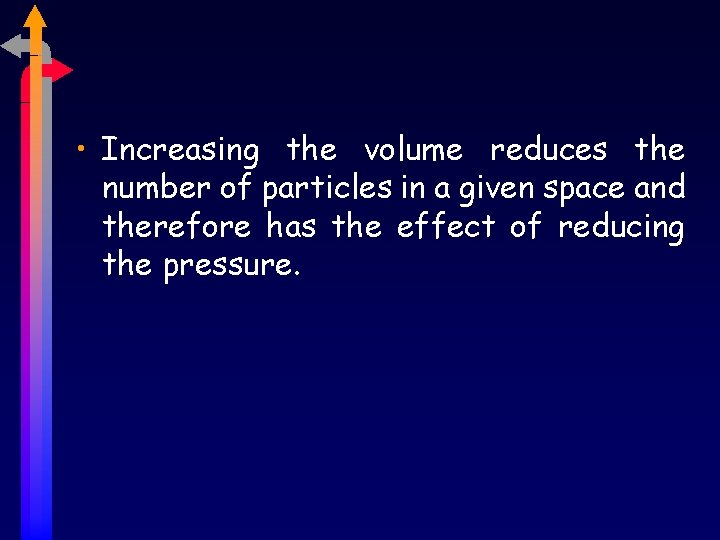
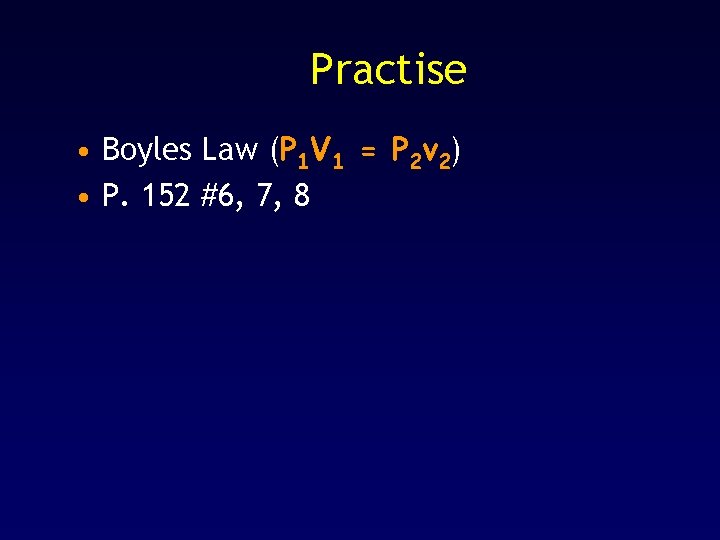
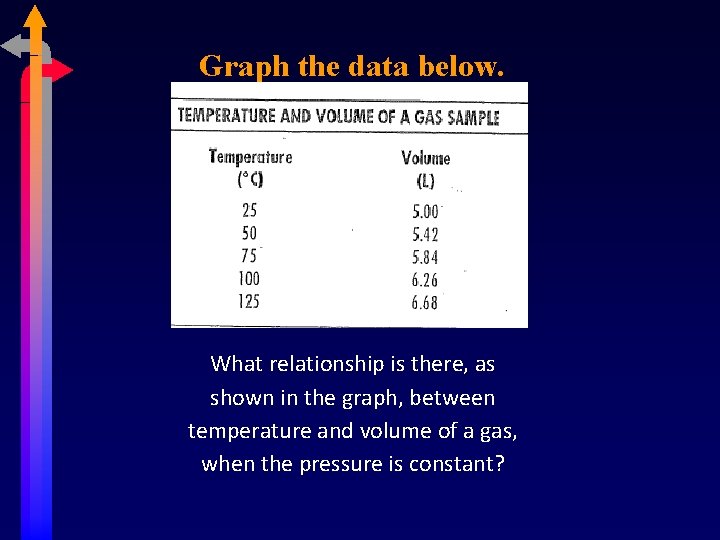
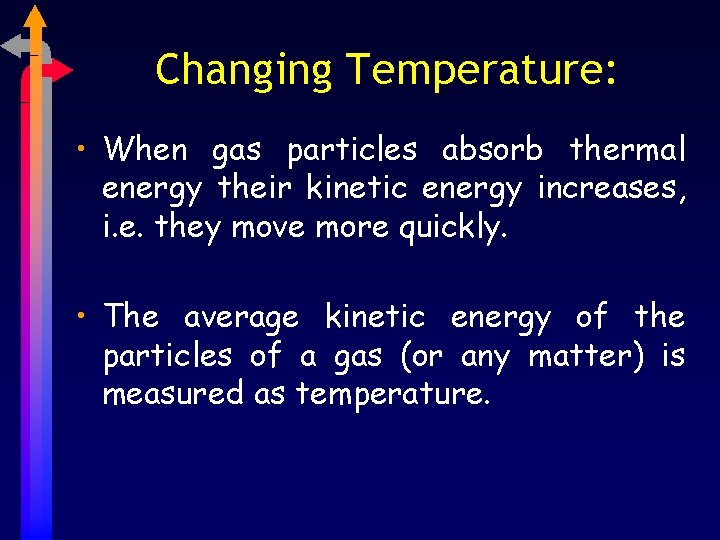
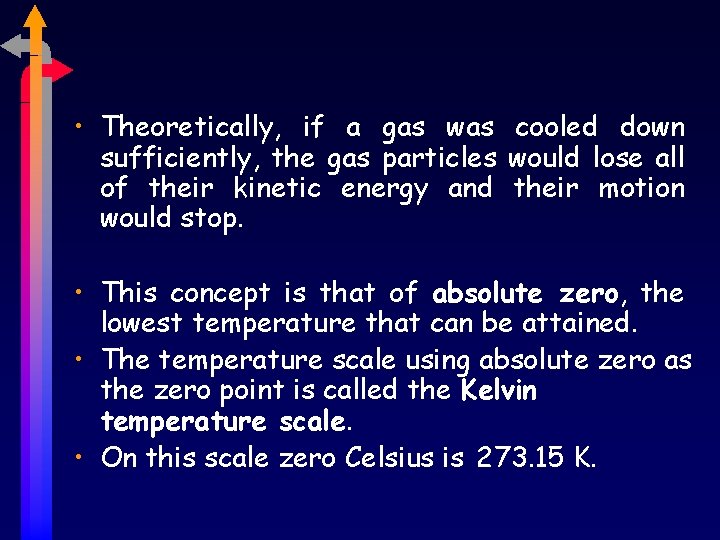
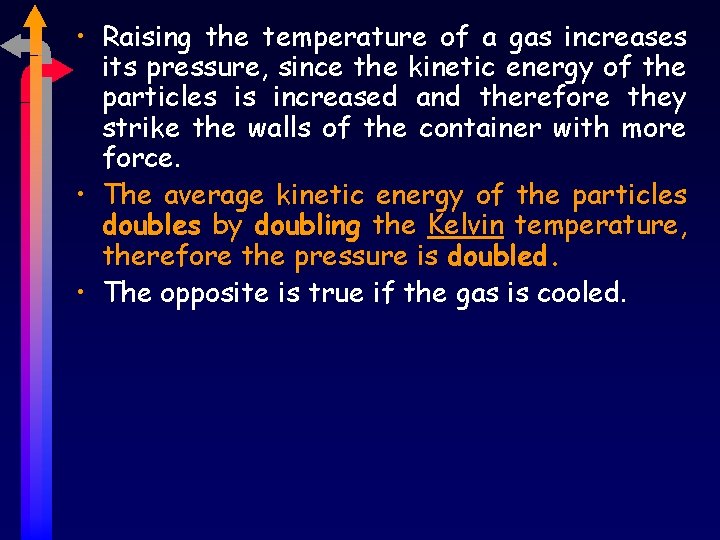
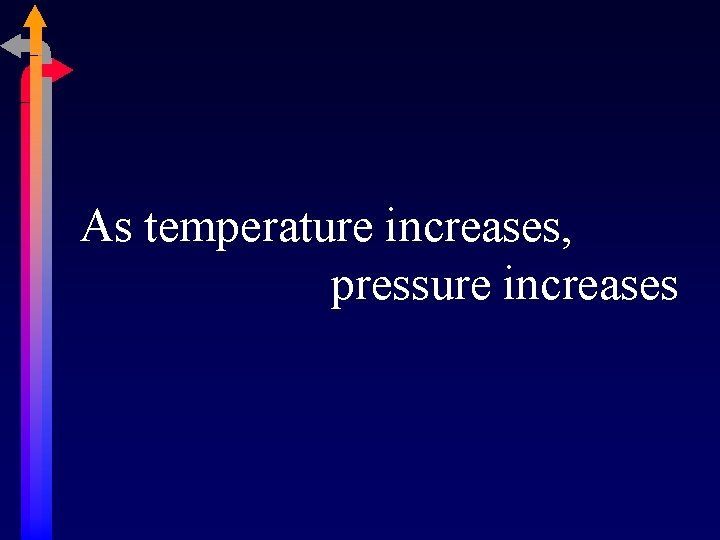
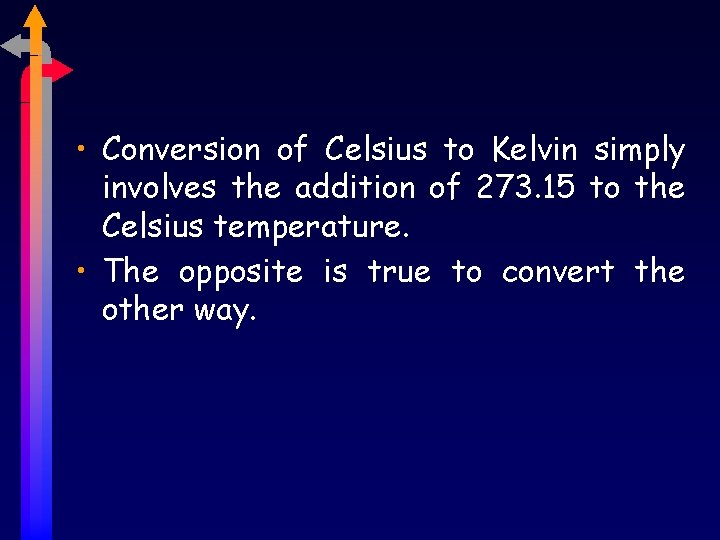
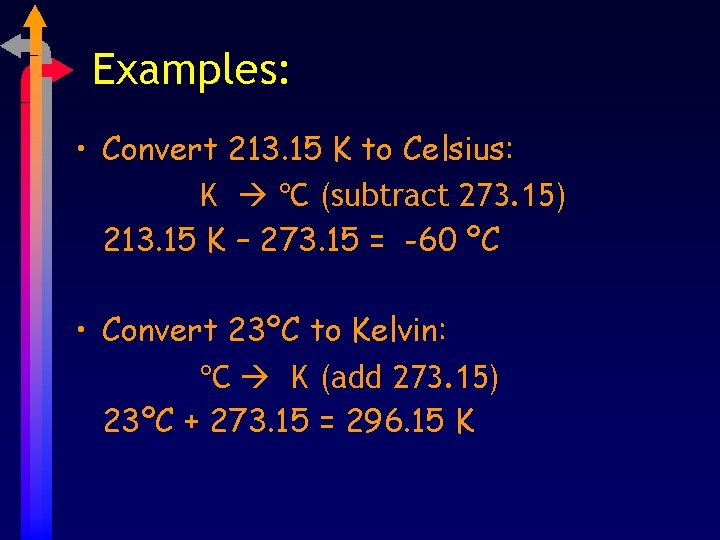
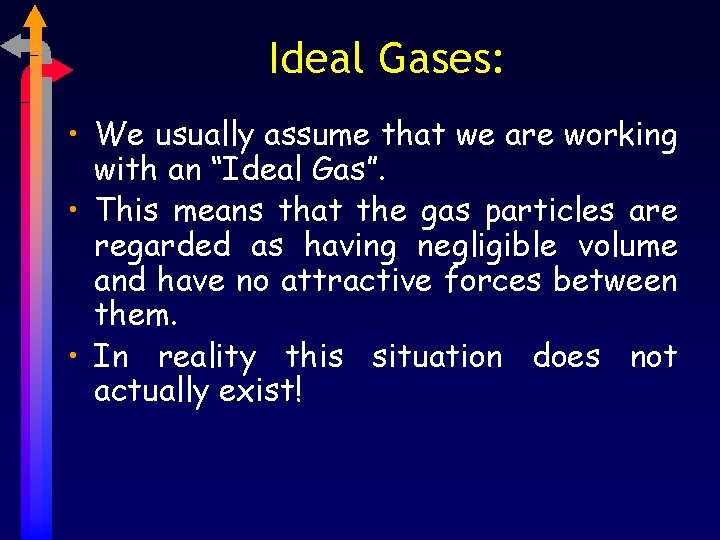
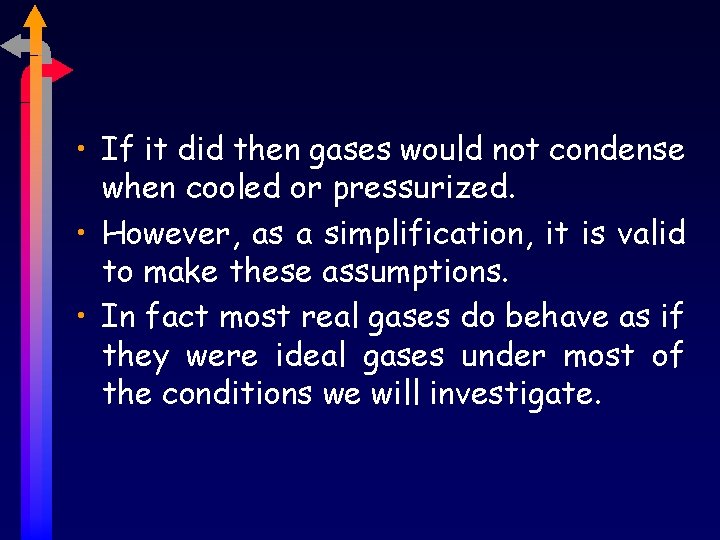
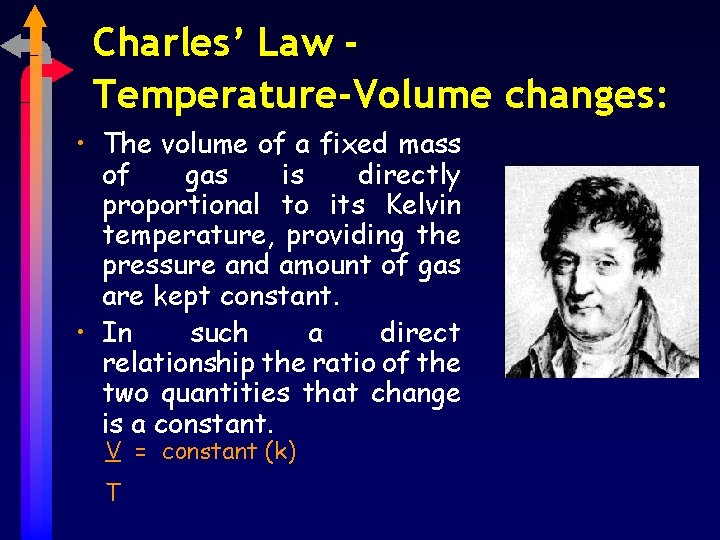
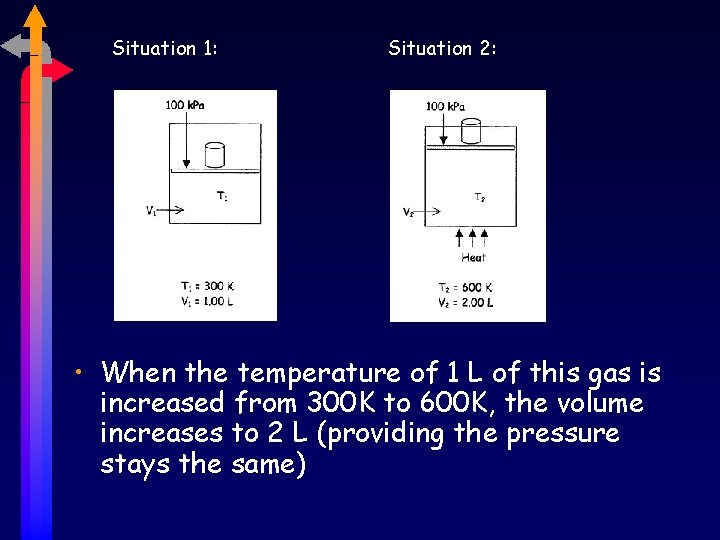
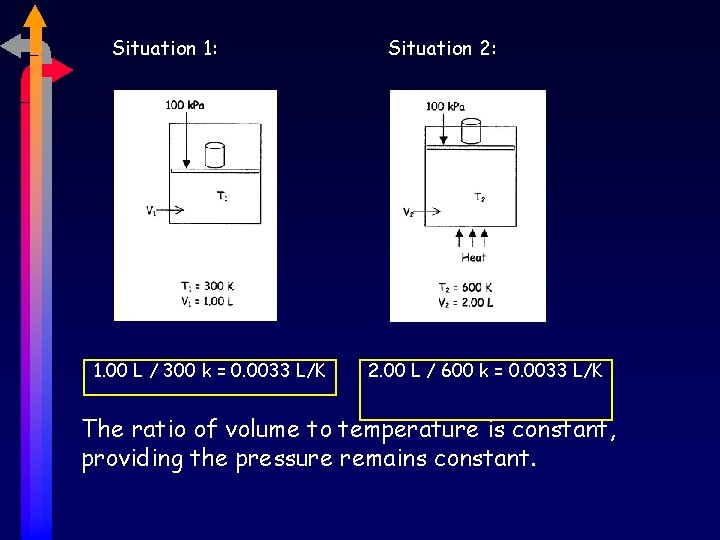
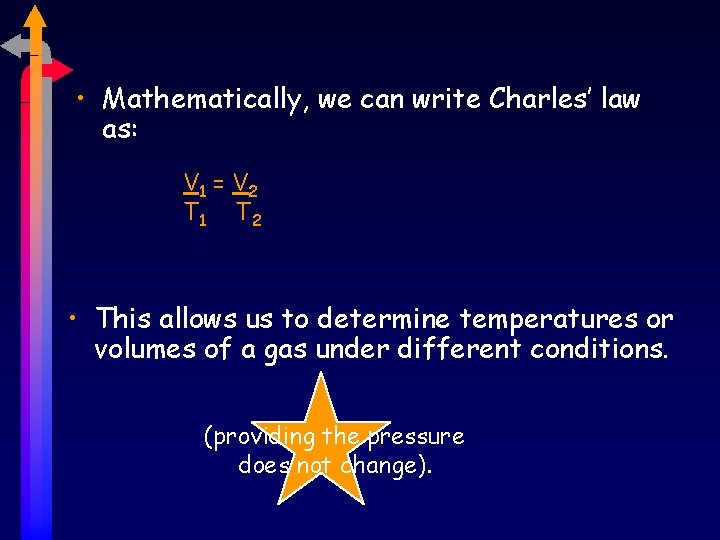
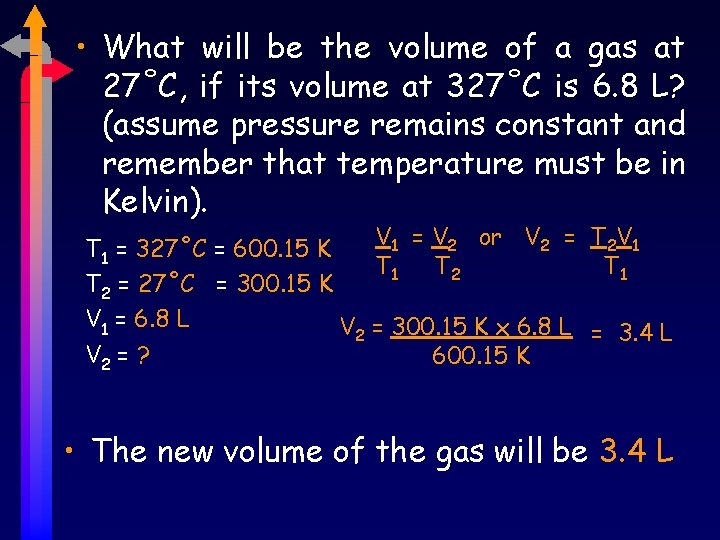
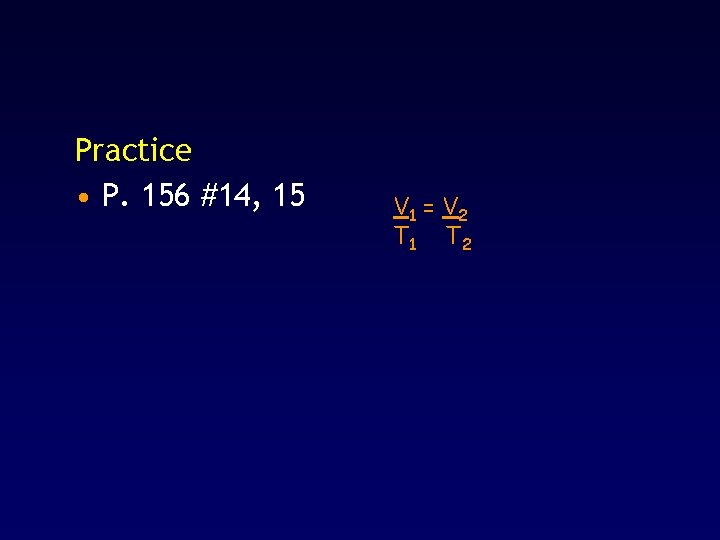
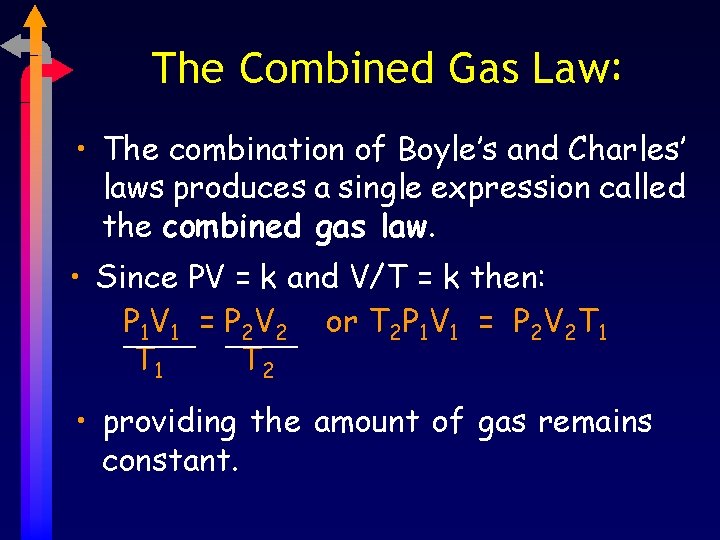
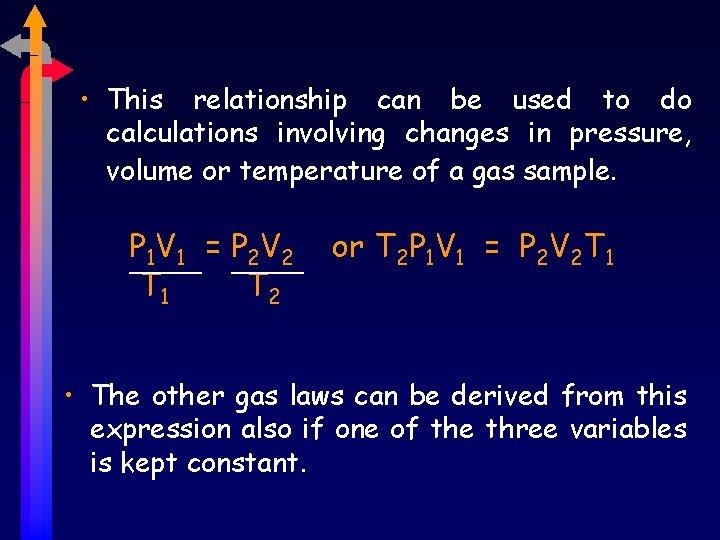
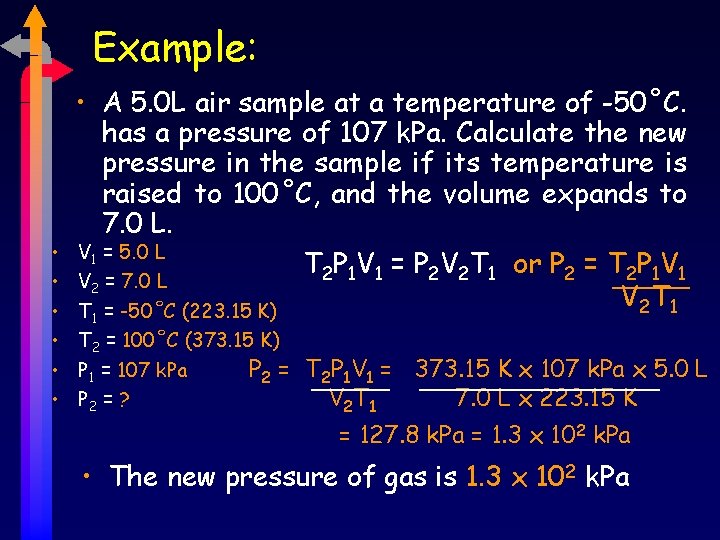
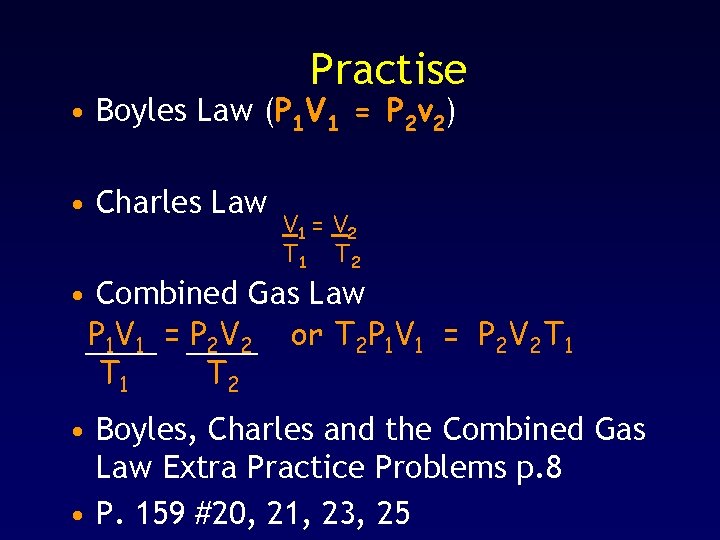
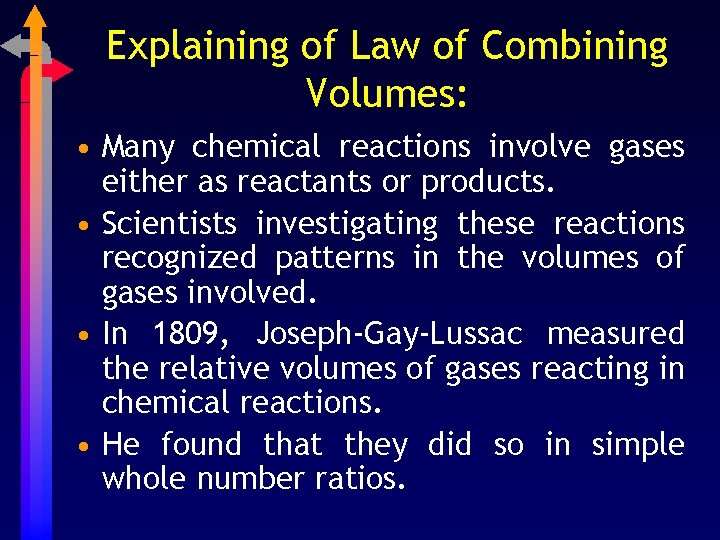
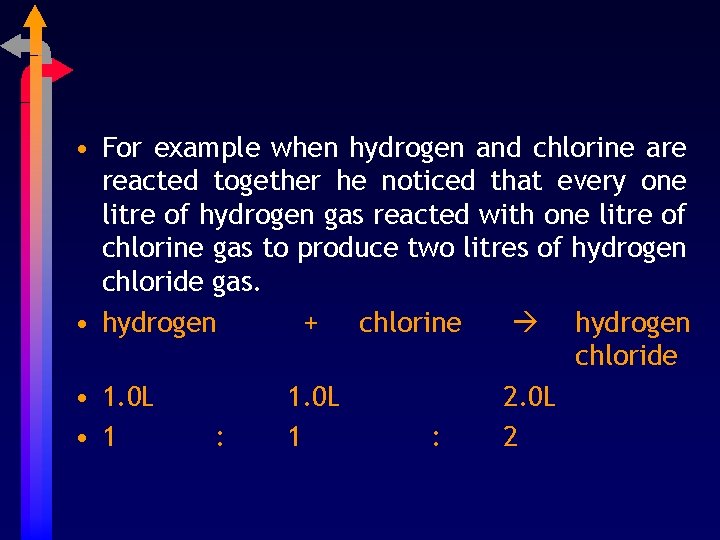
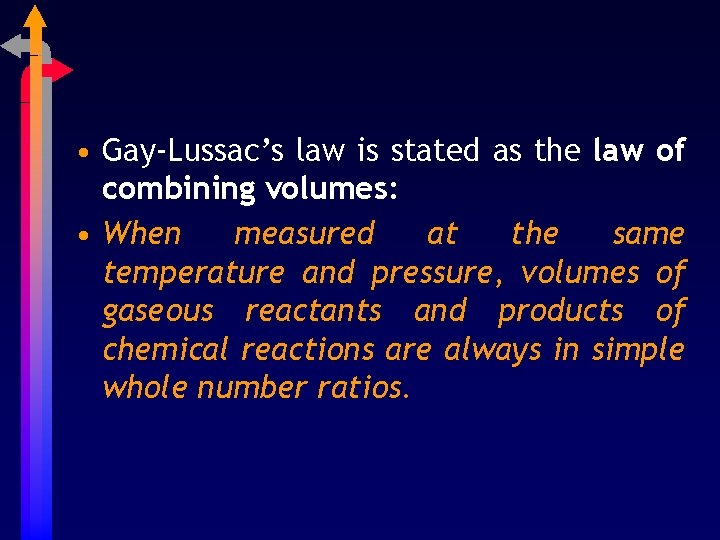
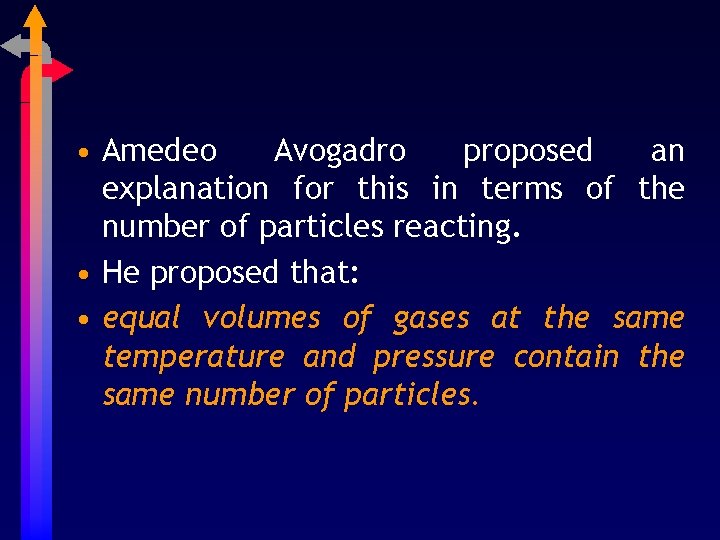
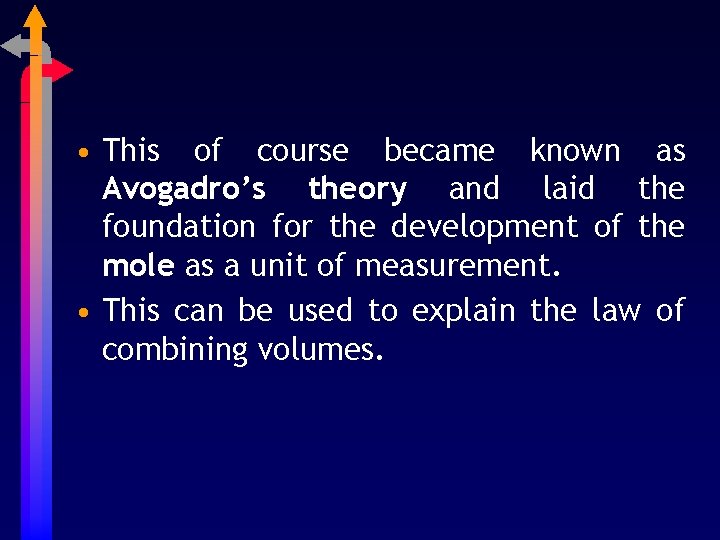
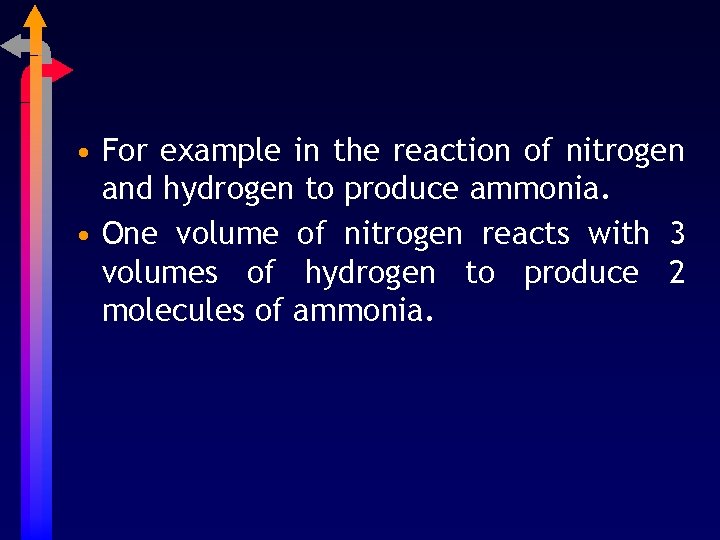
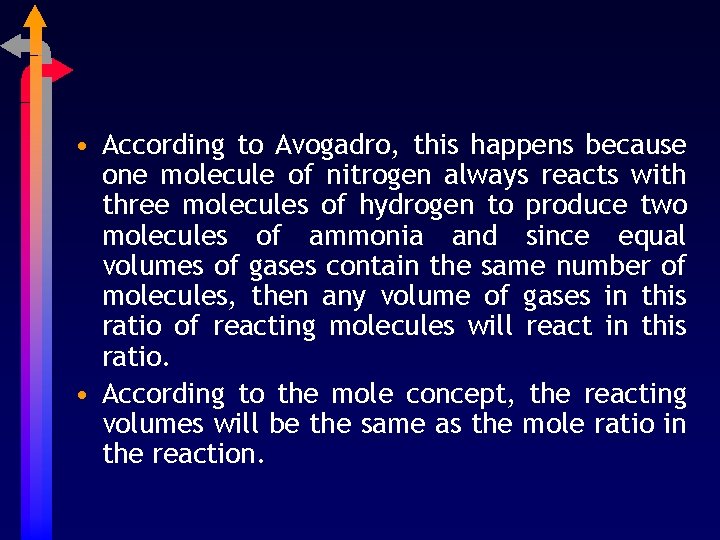
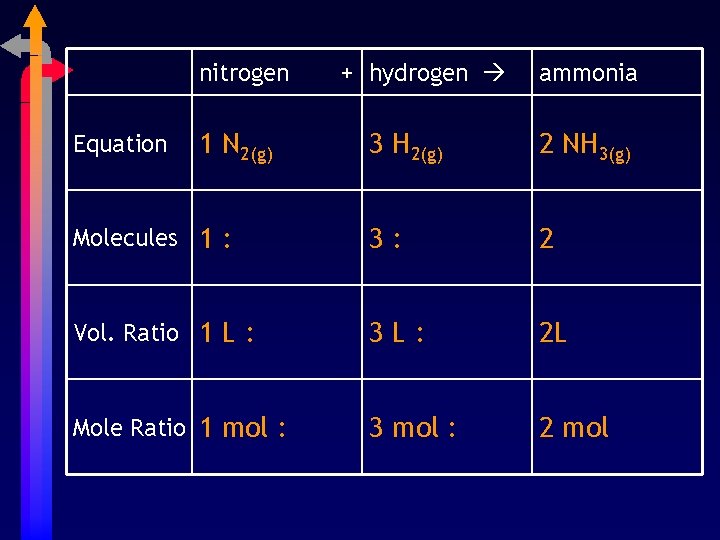
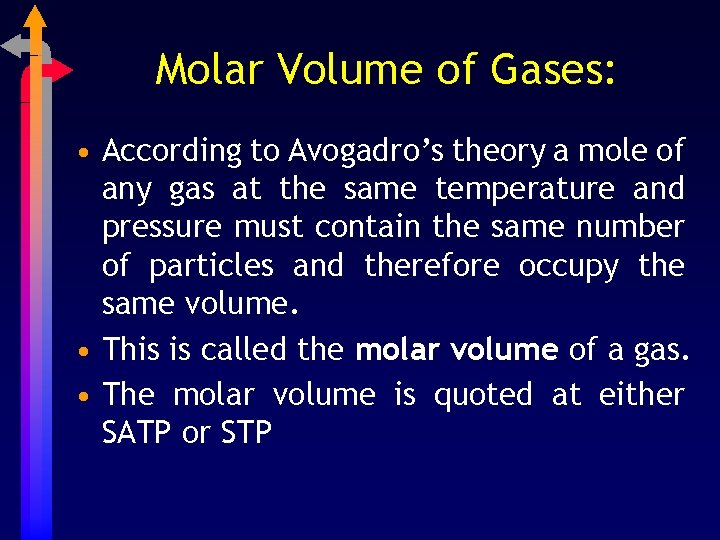
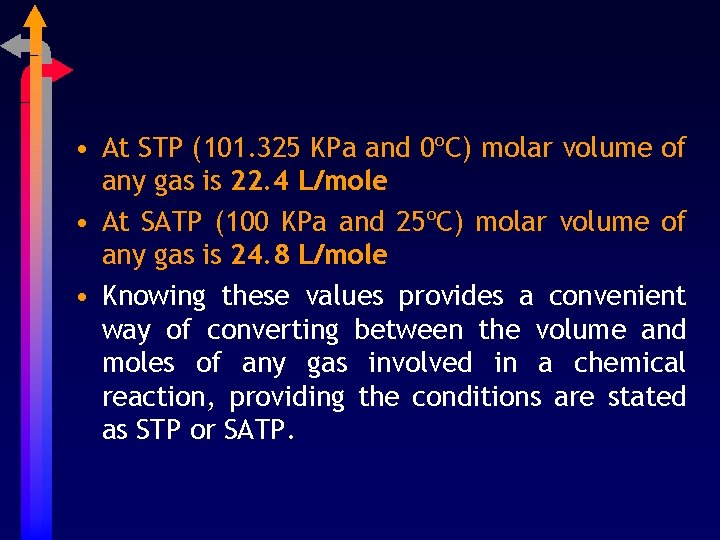
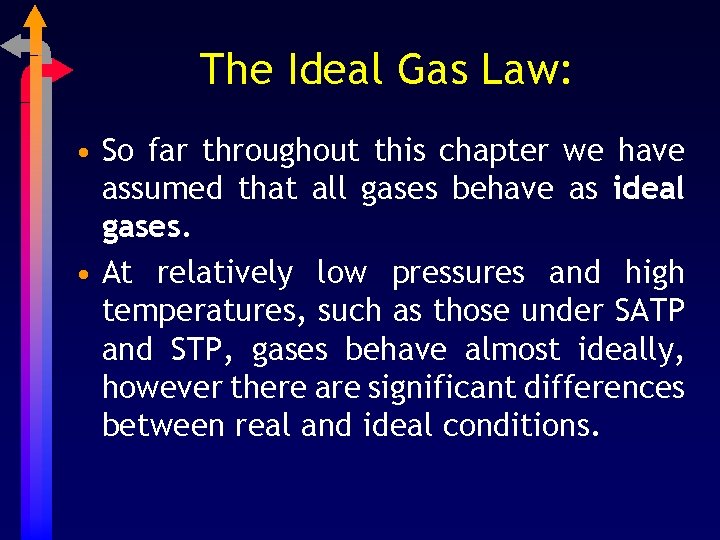
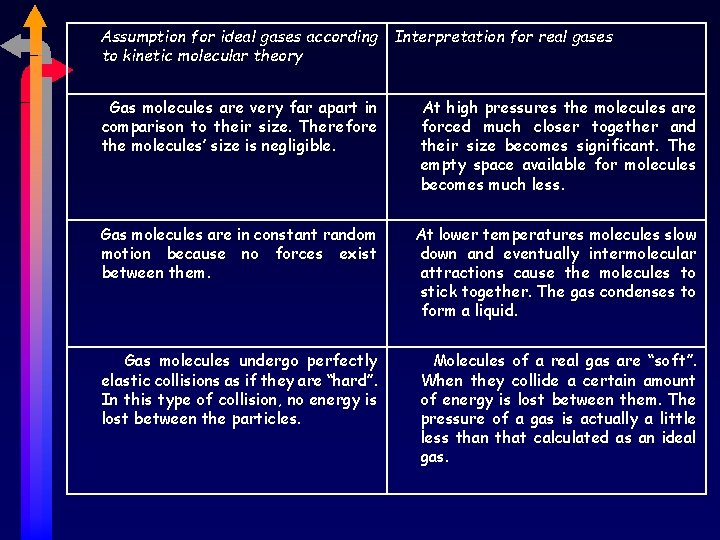
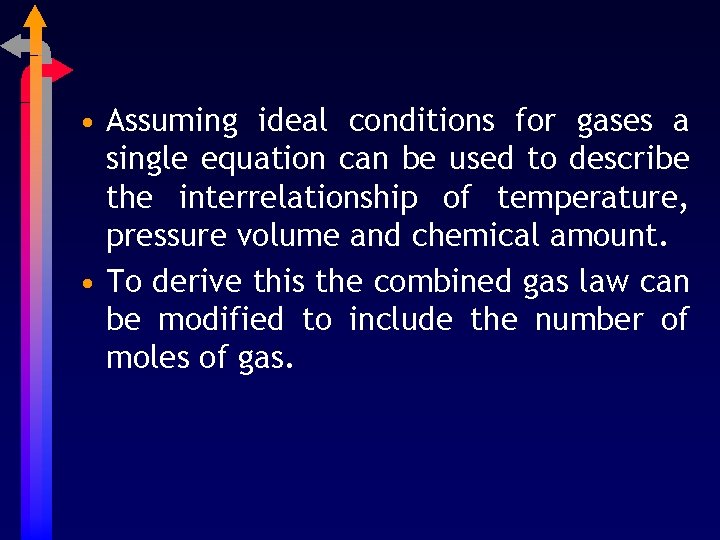
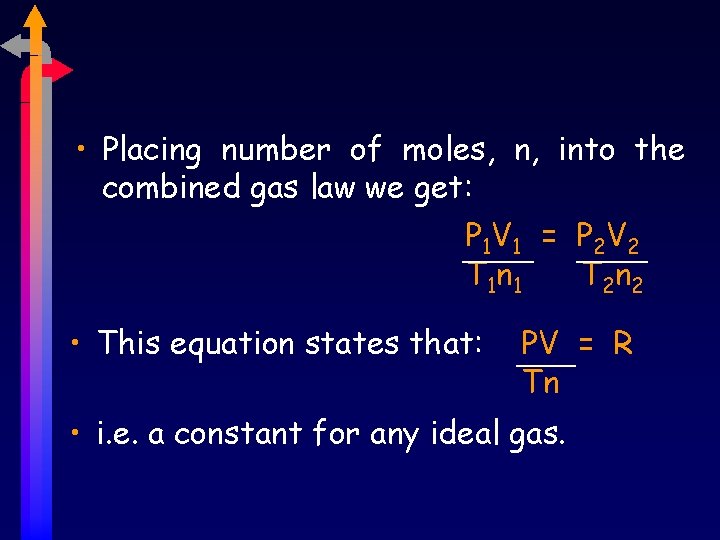
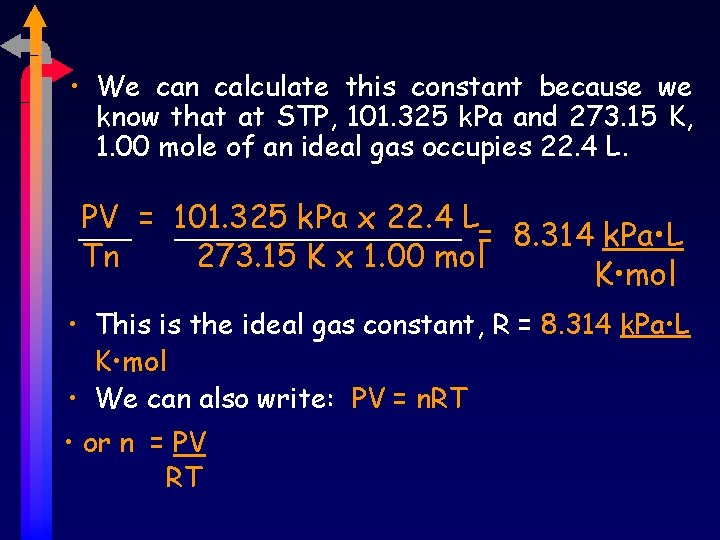
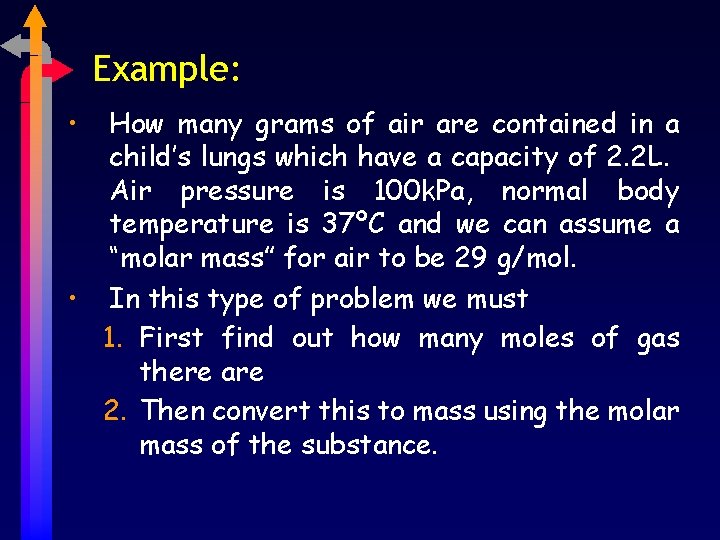
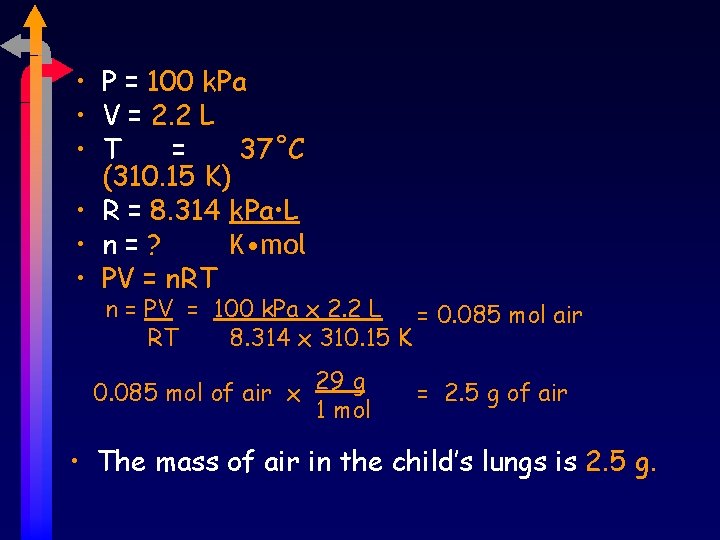
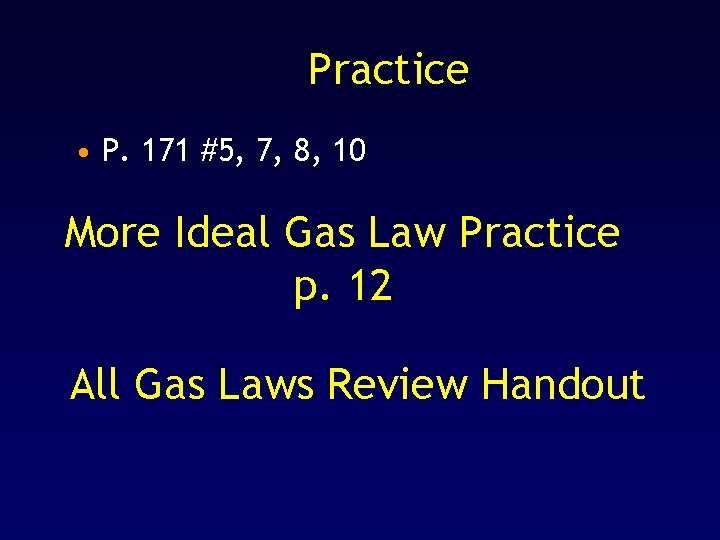
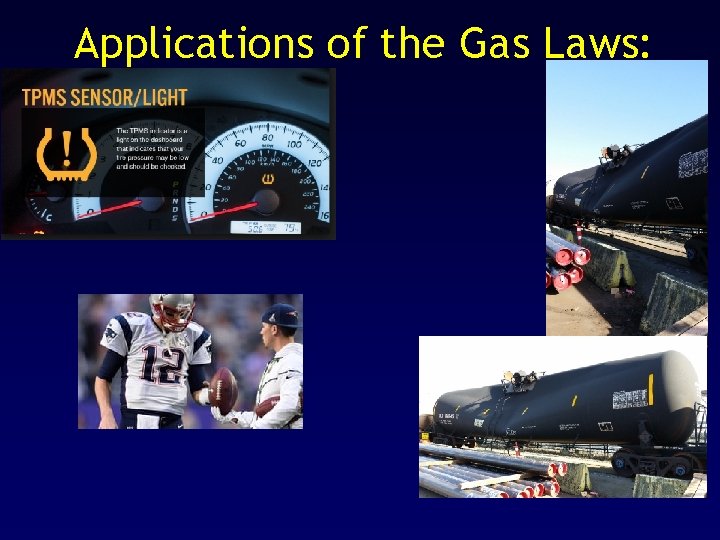
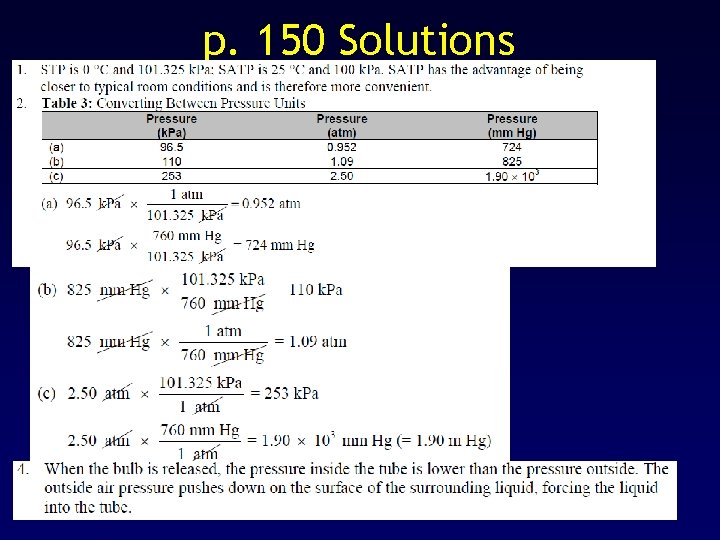
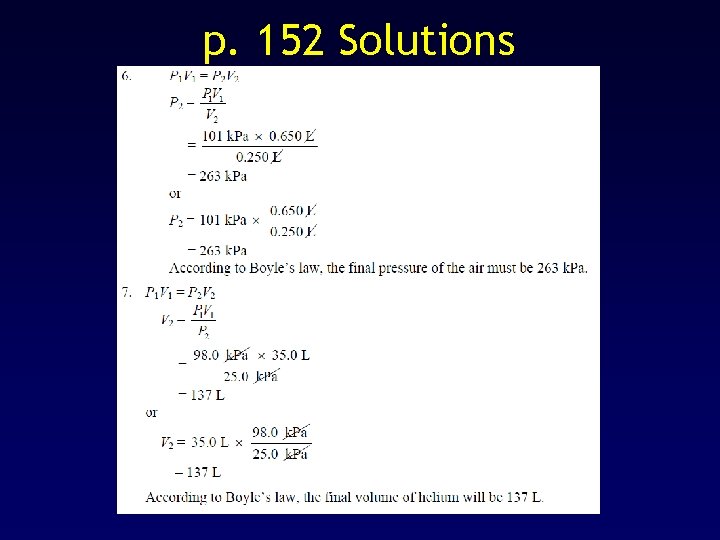
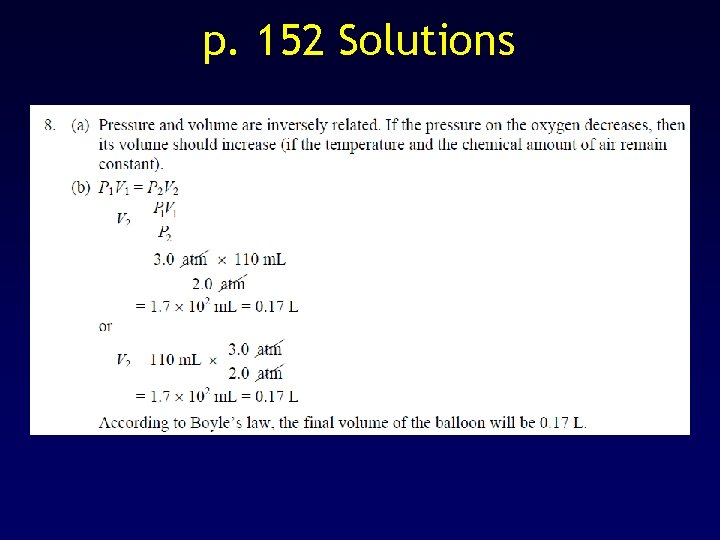
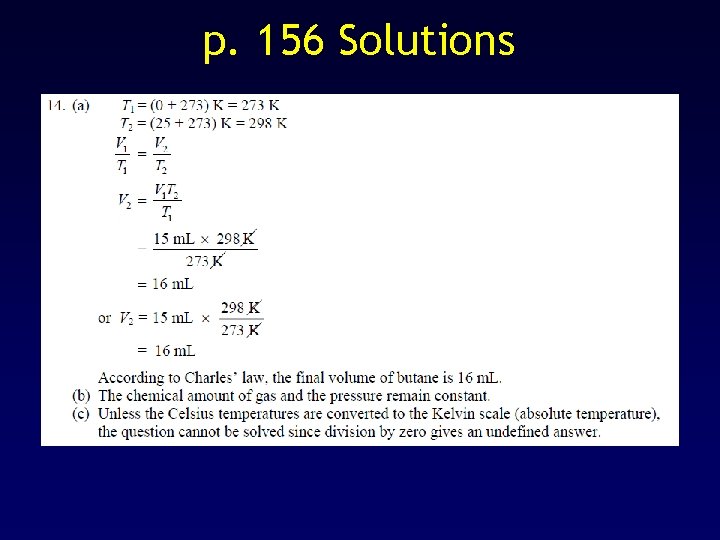
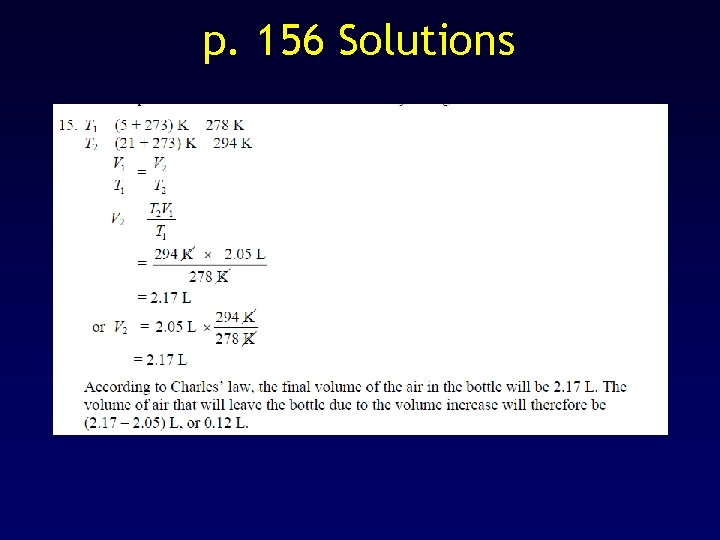
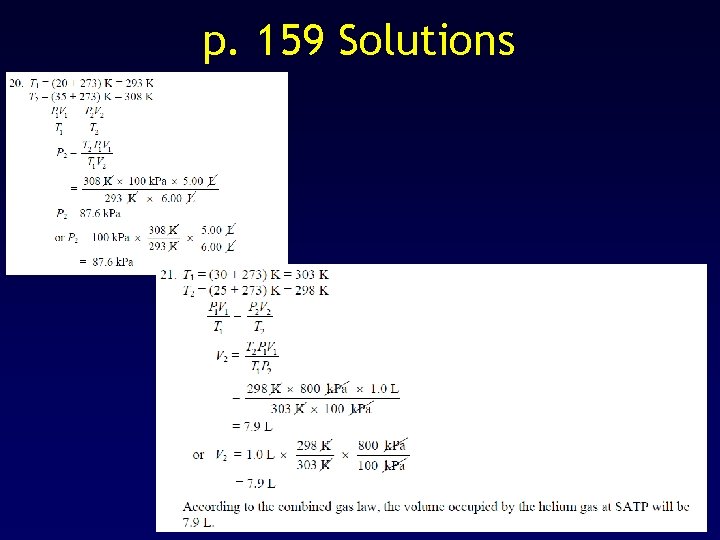
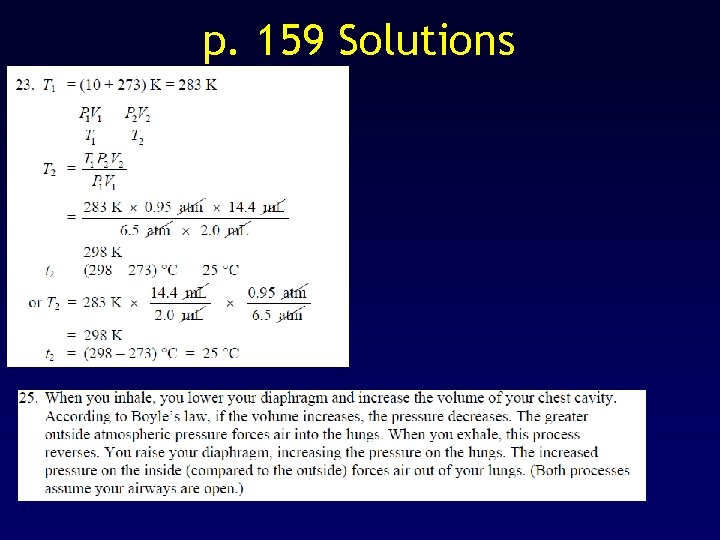
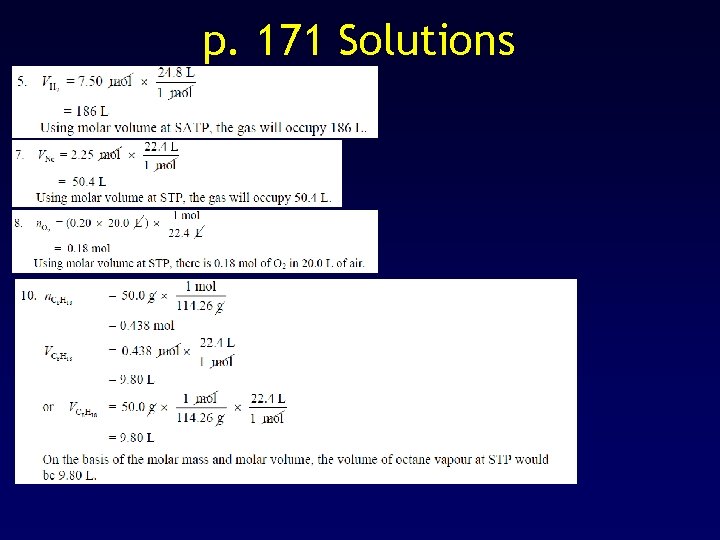
- Slides: 73
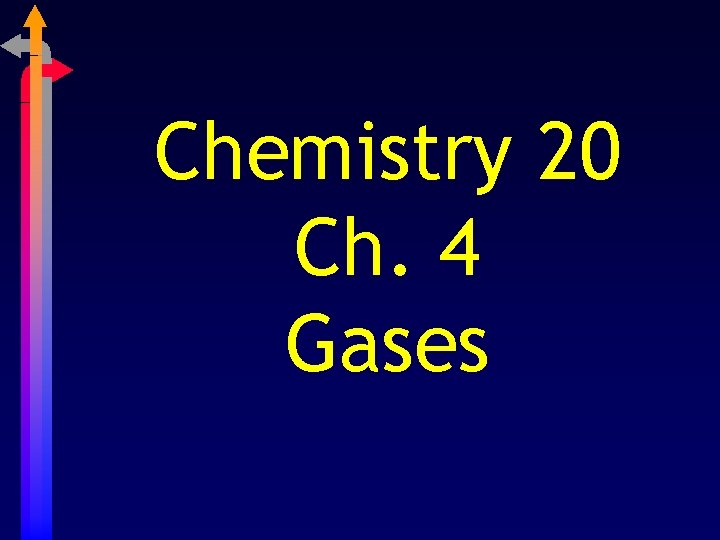
Chemistry 20 Ch. 4 Gases
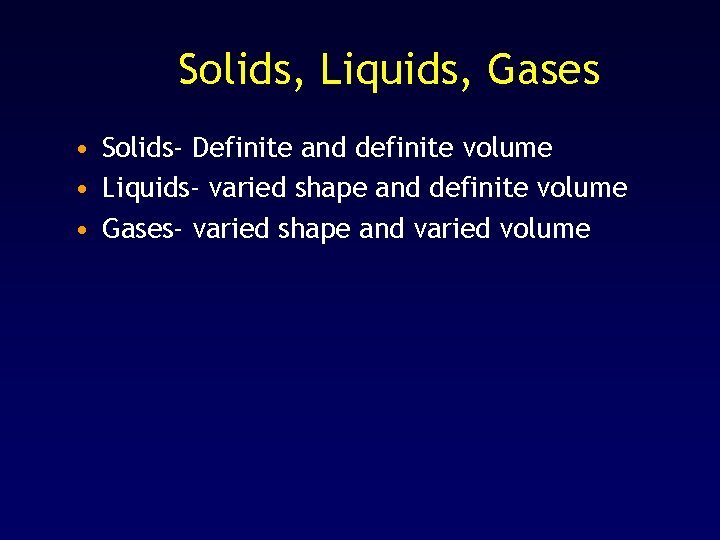
Solids, Liquids, Gases • Solids- Definite and definite volume • Liquids- varied shape and definite volume • Gases- varied shape and varied volume
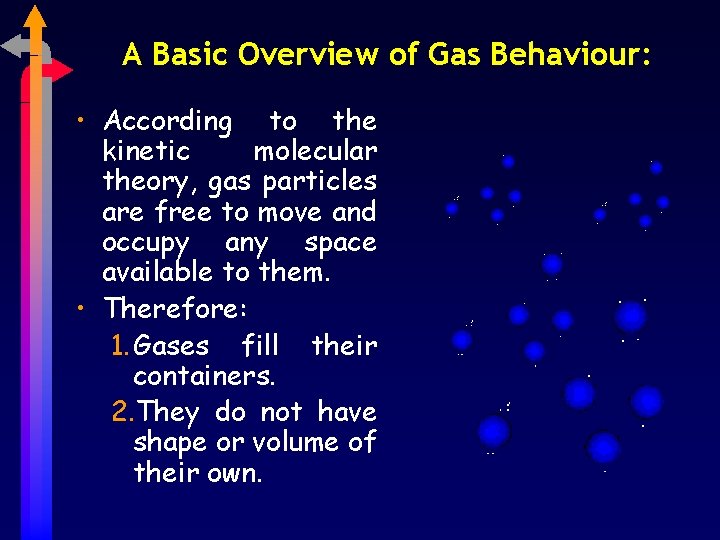
A Basic Overview of Gas Behaviour: • According to the kinetic molecular theory, gas particles are free to move and occupy any space available to them. • Therefore: 1. Gases fill their containers. 2. They do not have shape or volume of their own.
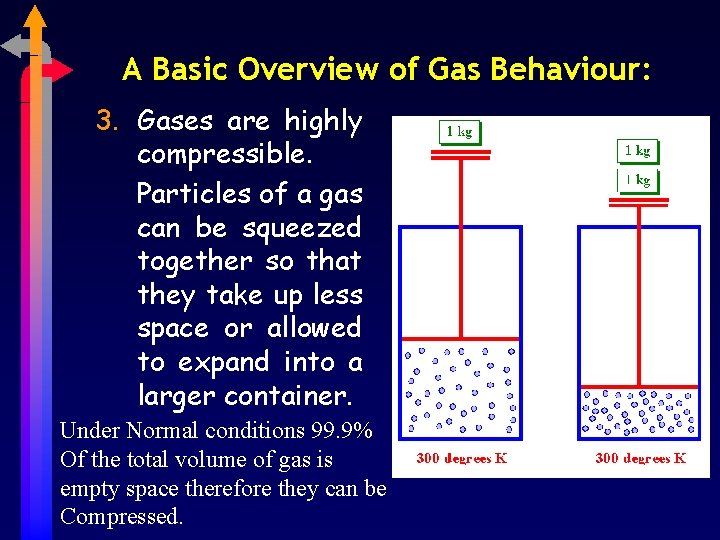
A Basic Overview of Gas Behaviour: 3. Gases are highly compressible. Particles of a gas can be squeezed together so that they take up less space or allowed to expand into a larger container. Under Normal conditions 99. 9% Of the total volume of gas is empty space therefore they can be Compressed.
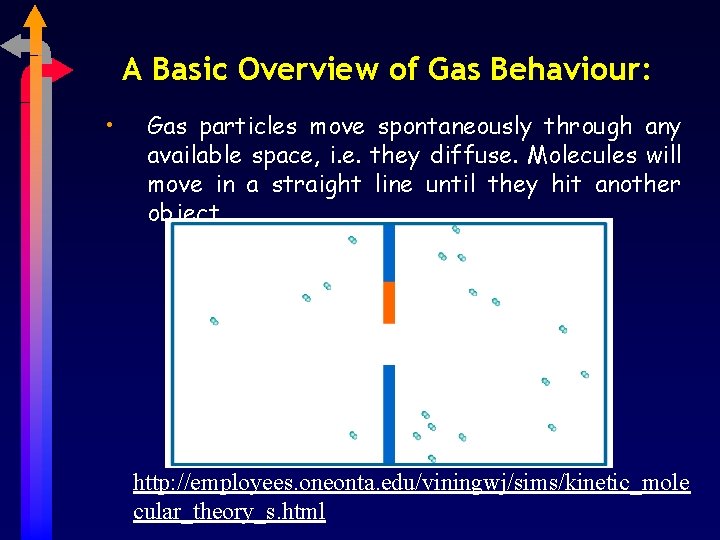
A Basic Overview of Gas Behaviour: • Gas particles move spontaneously through any available space, i. e. they diffuse. Molecules will move in a straight line until they hit another object. http: //employees. oneonta. edu/viningwj/sims/kinetic_mole cular_theory_s. html
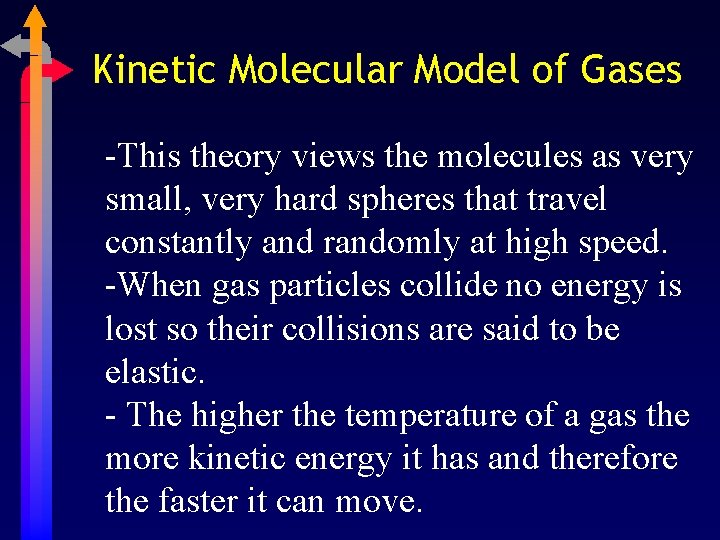
Kinetic Molecular Model of Gases -This theory views the molecules as very small, very hard spheres that travel constantly and randomly at high speed. -When gas particles collide no energy is lost so their collisions are said to be elastic. - The higher the temperature of a gas the more kinetic energy it has and therefore the faster it can move.
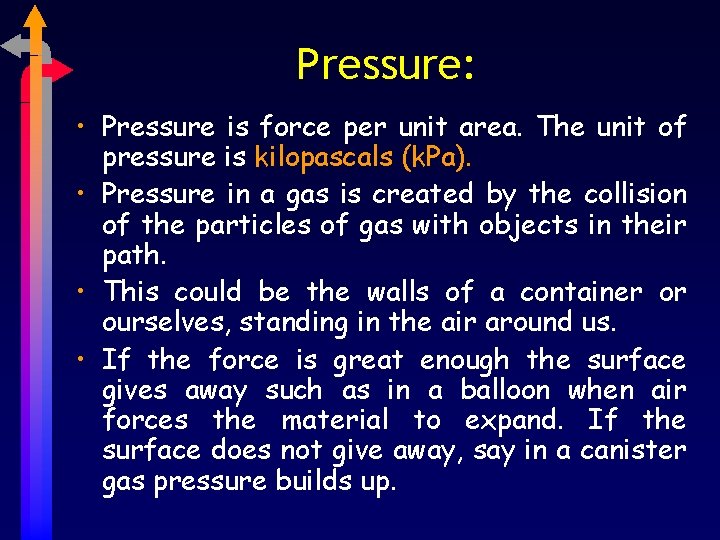
Pressure: • Pressure is force per unit area. The unit of pressure is kilopascals (k. Pa). • Pressure in a gas is created by the collision of the particles of gas with objects in their path. • This could be the walls of a container or ourselves, standing in the air around us. • If the force is great enough the surface gives away such as in a balloon when air forces the material to expand. If the surface does not give away, say in a canister gas pressure builds up.
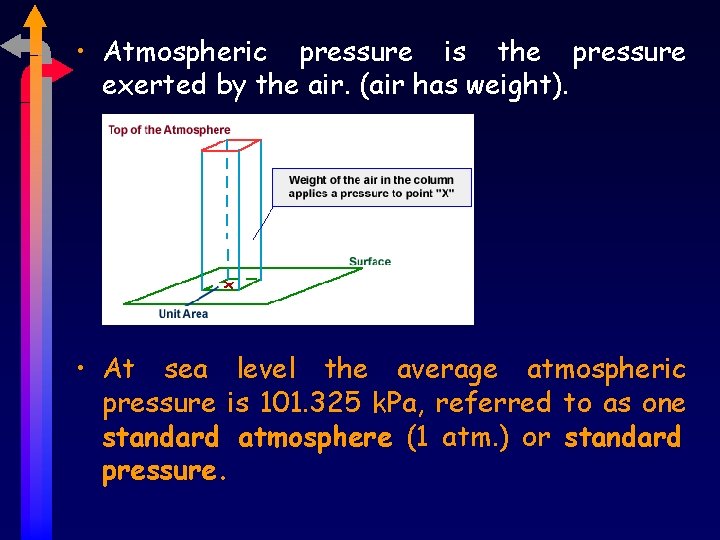
• Atmospheric pressure is the pressure exerted by the air. (air has weight). • At sea level the average atmospheric pressure is 101. 325 k. Pa, referred to as one standard atmosphere (1 atm. ) or standard pressure.
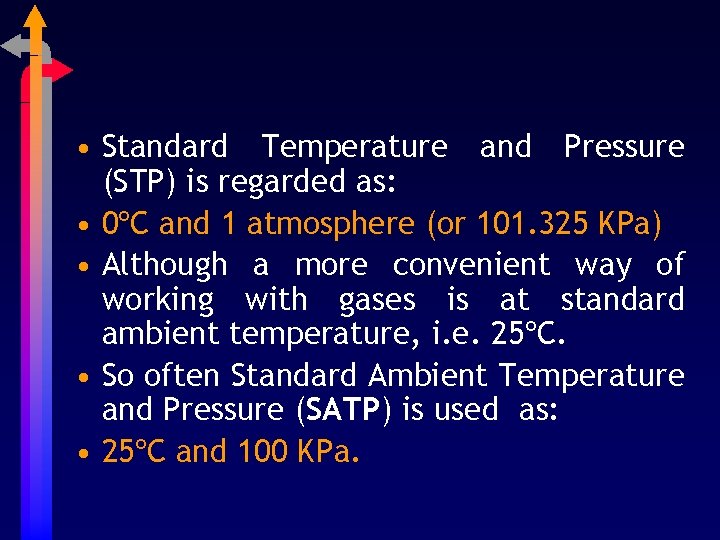
• Standard Temperature and Pressure (STP) is regarded as: • 0ºC and 1 atmosphere (or 101. 325 KPa) • Although a more convenient way of working with gases is at standard ambient temperature, i. e. 25ºC. • So often Standard Ambient Temperature and Pressure (SATP) is used as: • 25ºC and 100 KPa.
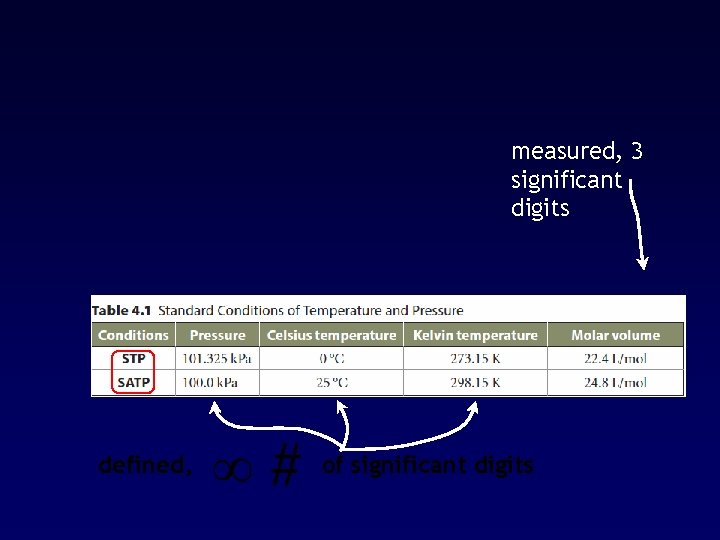
measured, 3 significant digits defined, of significant digits
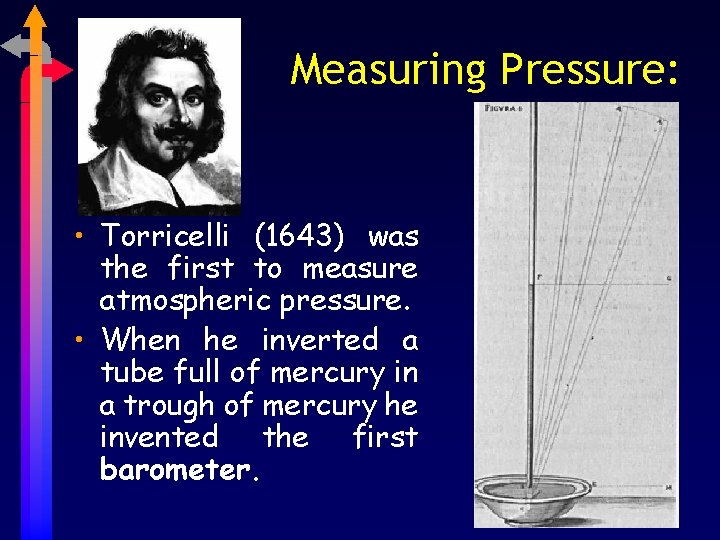
Measuring Pressure: • Torricelli (1643) was the first to measure atmospheric pressure. • When he inverted a tube full of mercury in a trough of mercury he invented the first barometer.
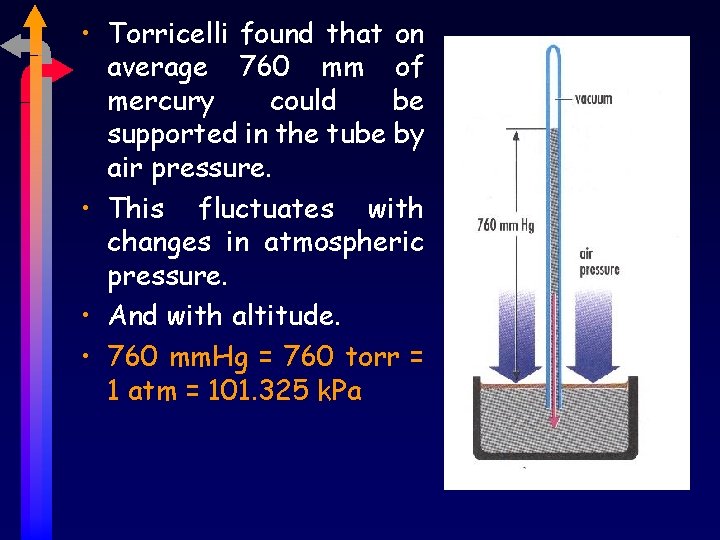
• Torricelli found that on average 760 mm of mercury could be supported in the tube by air pressure. • This fluctuates with changes in atmospheric pressure. • And with altitude. • 760 mm. Hg = 760 torr = 1 atm = 101. 325 k. Pa
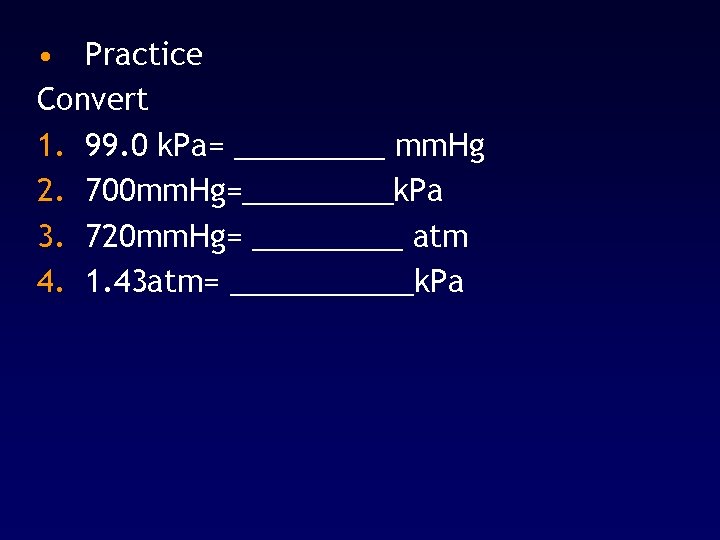
• Practice Convert 1. 99. 0 k. Pa= _____ mm. Hg 2. 700 mm. Hg=_____k. Pa 3. 720 mm. Hg= _____ atm 4. 1. 43 atm= ______k. Pa
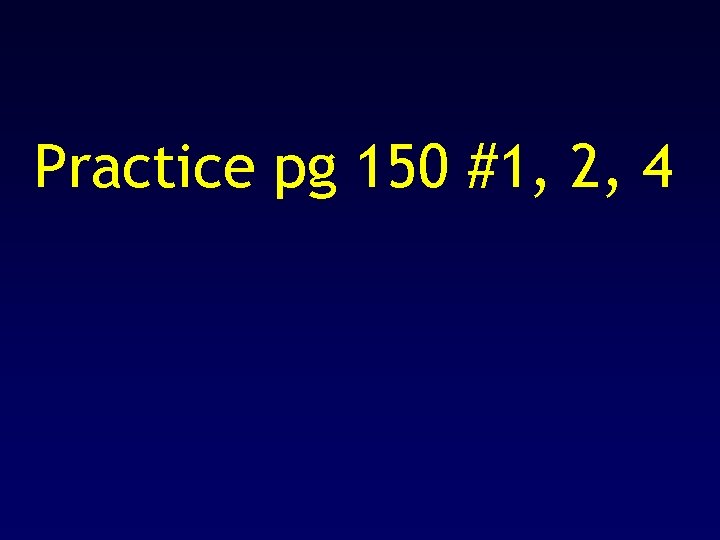
Practice pg 150 #1, 2, 4
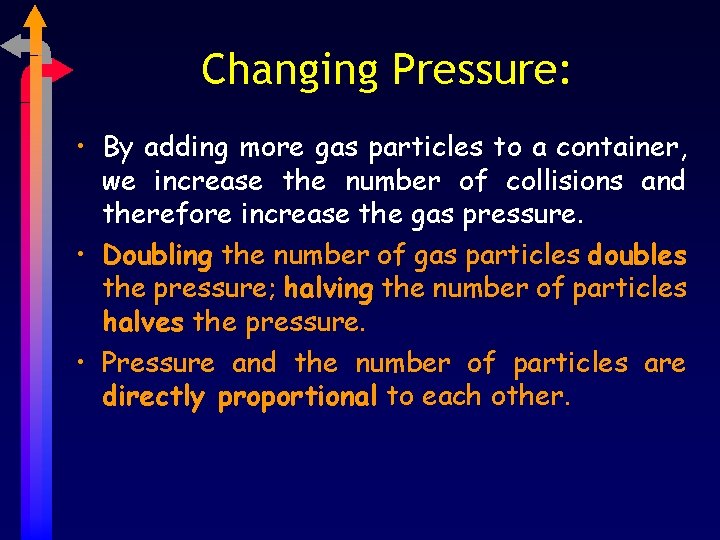
Changing Pressure: • By adding more gas particles to a container, we increase the number of collisions and therefore increase the gas pressure. • Doubling the number of gas particles doubles the pressure; halving the number of particles halves the pressure. • Pressure and the number of particles are directly proportional to each other.
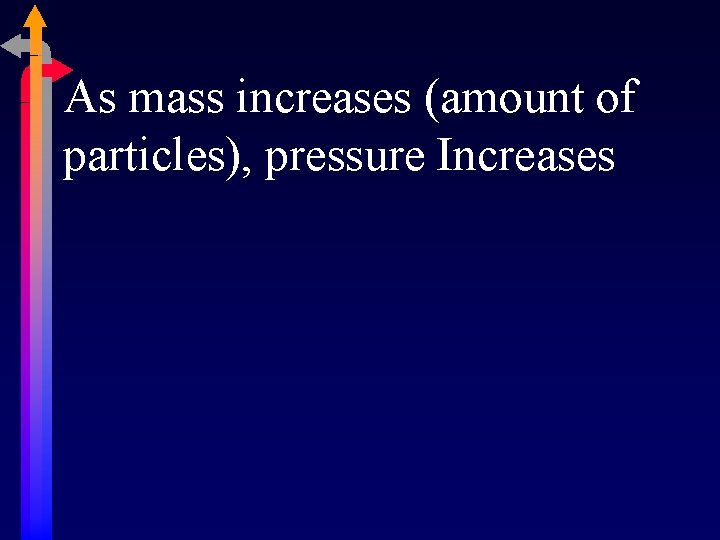
As mass increases (amount of particles), pressure Increases
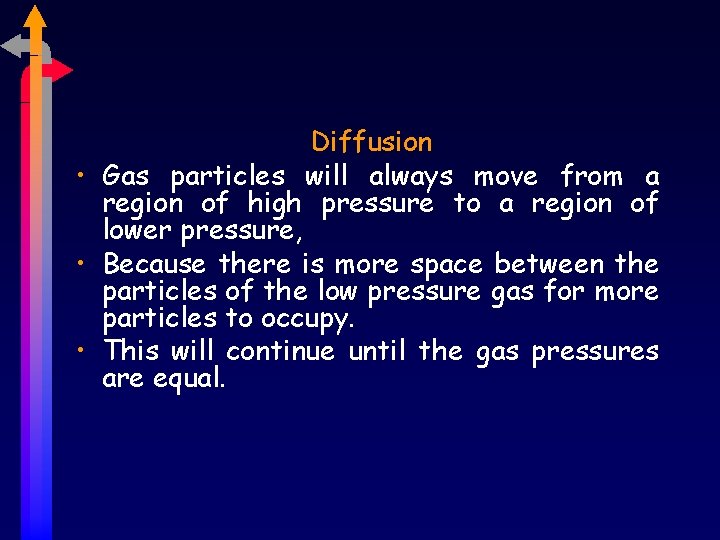
Diffusion • Gas particles will always move from a region of high pressure to a region of lower pressure, • Because there is more space between the particles of the low pressure gas for more particles to occupy. • This will continue until the gas pressures are equal.
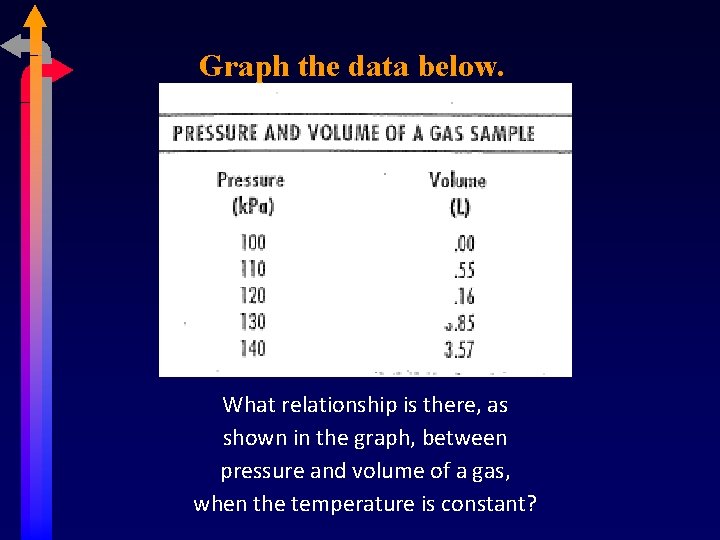
Graph the data below. What relationship is there, as shown in the graph, between pressure and volume of a gas, when the temperature is constant?
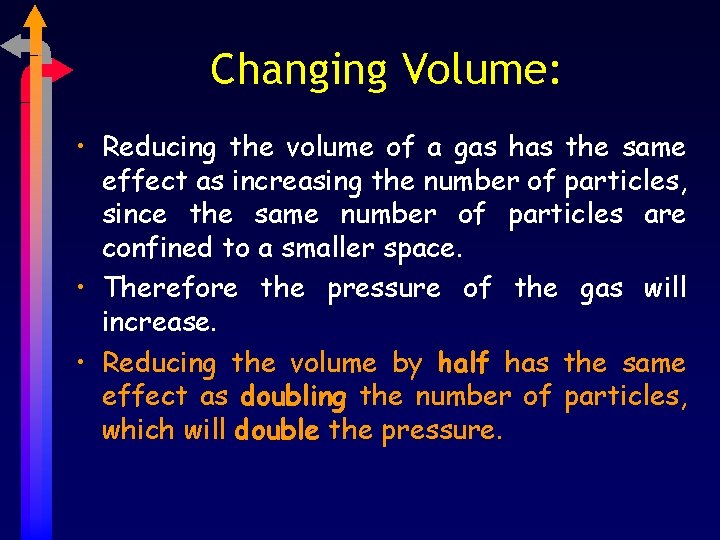
Changing Volume: • Reducing the volume of a gas has the same effect as increasing the number of particles, since the same number of particles are confined to a smaller space. • Therefore the pressure of the gas will increase. • Reducing the volume by half has the same effect as doubling the number of particles, which will double the pressure.
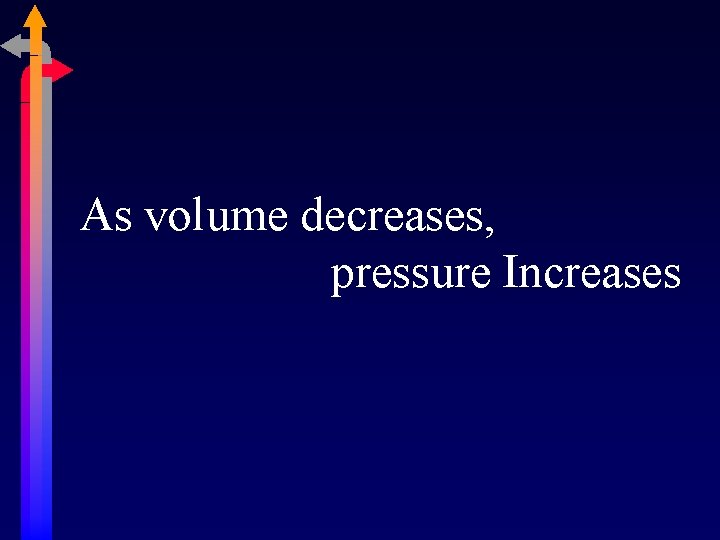
As volume decreases, pressure Increases
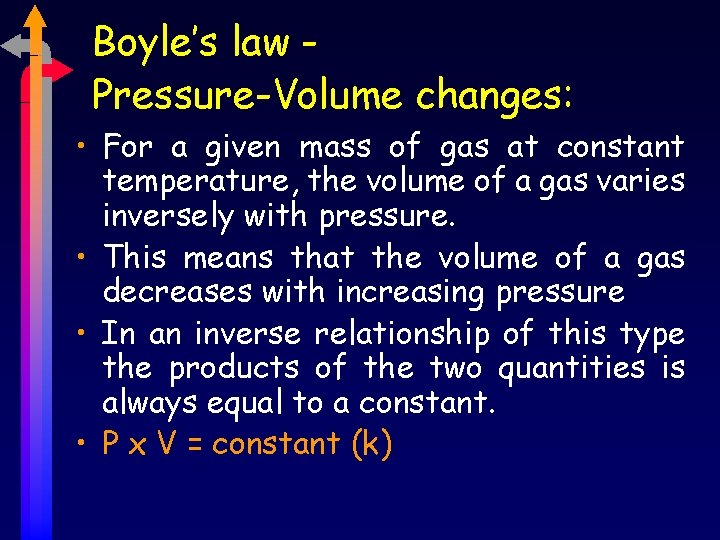
Boyle’s law Pressure-Volume changes: • For a given mass of gas at constant temperature, the volume of a gas varies inversely with pressure. • This means that the volume of a gas decreases with increasing pressure • In an inverse relationship of this type the products of the two quantities is always equal to a constant. • P x V = constant (k)
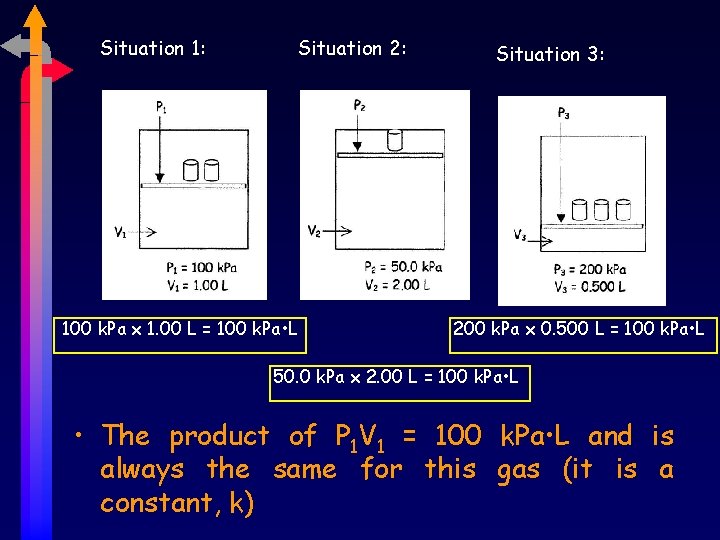
Situation 1: Situation 2: 100 k. Pa x 1. 00 L = 100 k. Pa • L Situation 3: 200 k. Pa x 0. 500 L = 100 k. Pa • L 50. 0 k. Pa x 2. 00 L = 100 k. Pa • L • The product of P 1 V 1 = 100 k. Pa • L and is always the same for this gas (it is a constant, k)
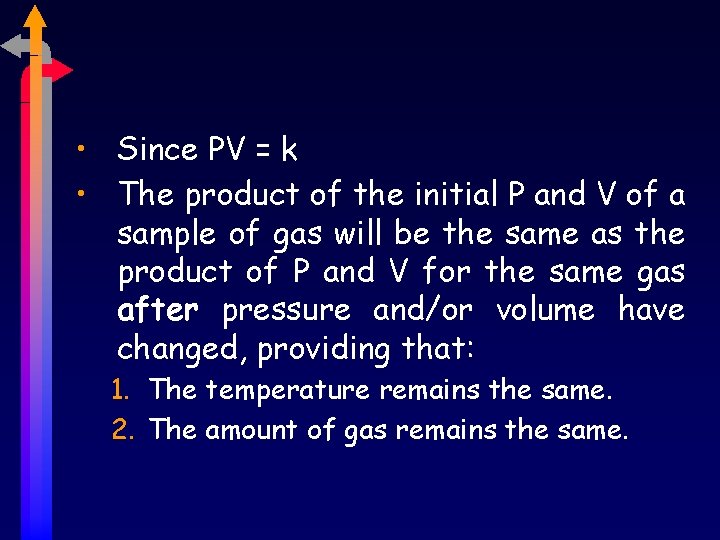
• Since PV = k • The product of the initial P and V of a sample of gas will be the same as the product of P and V for the same gas after pressure and/or volume have changed, providing that: 1. The temperature remains the same. 2. The amount of gas remains the same.
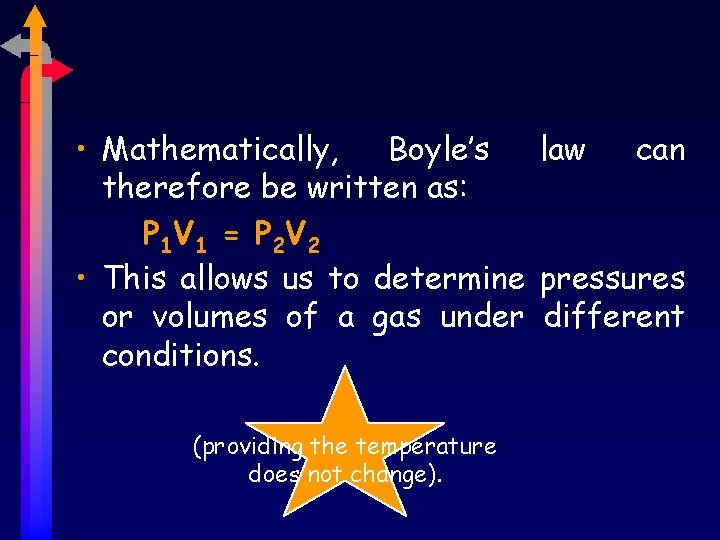
• Mathematically, Boyle’s law can therefore be written as: P 1 V 1 = P 2 V 2 • This allows us to determine pressures or volumes of a gas under different conditions. (providing the temperature does not change).
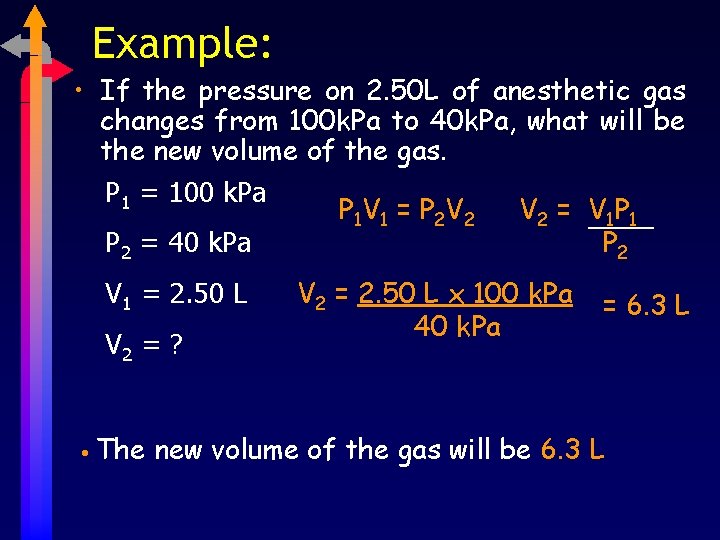
Example: • If the pressure on 2. 50 L of anesthetic gas changes from 100 k. Pa to 40 k. Pa, what will be the new volume of the gas. P 1 = 100 k. Pa P 1 V 1 = P 2 V 2 = V 1 P 1 P 2 = 40 k. Pa V 1 = 2. 50 L V 2 = ? • V 2 = 2. 50 L x 100 k. Pa 40 k. Pa = 6. 3 L The new volume of the gas will be 6. 3 L
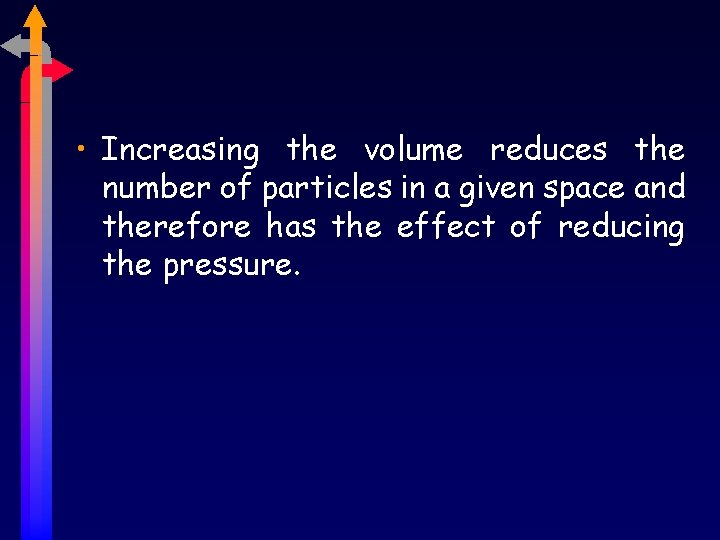
• Increasing the volume reduces the number of particles in a given space and therefore has the effect of reducing the pressure.
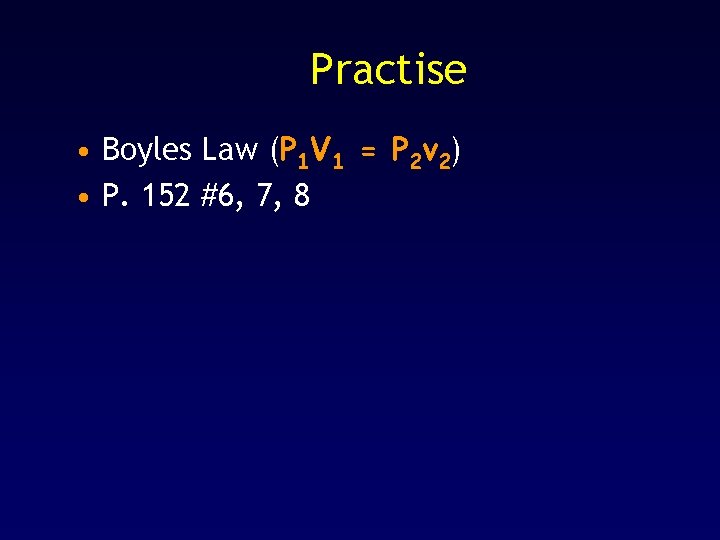
Practise • Boyles Law (P 1 V 1 = P 2 v 2) • P. 152 #6, 7, 8
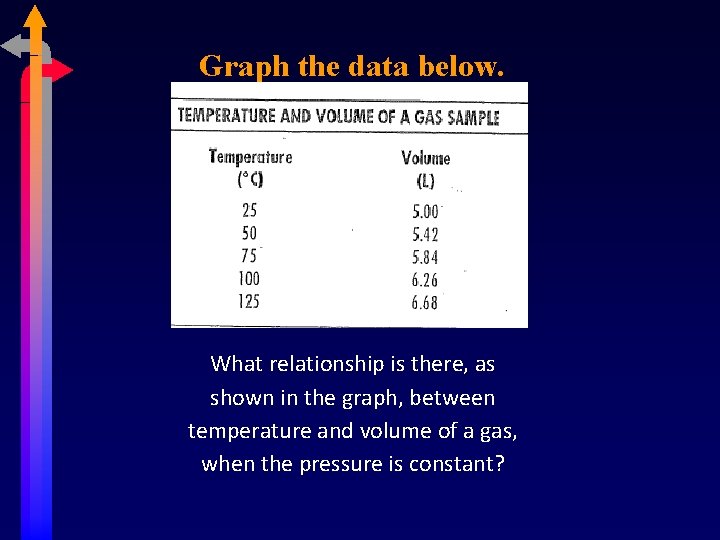
Graph the data below. What relationship is there, as shown in the graph, between temperature and volume of a gas, when the pressure is constant?
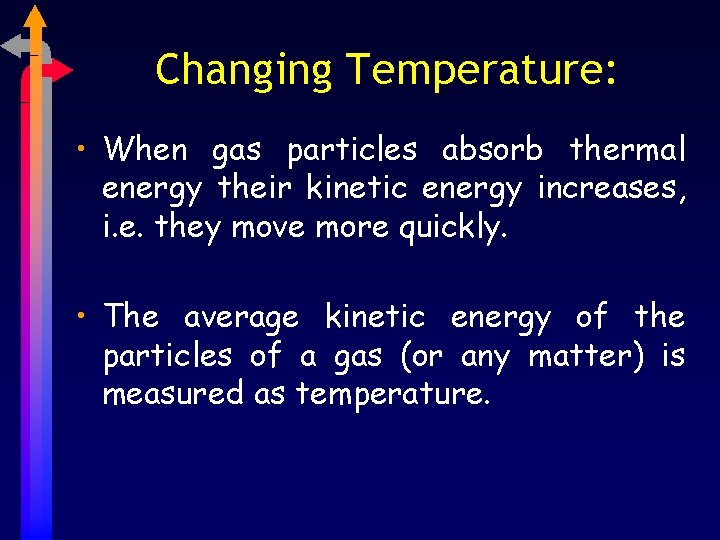
Changing Temperature: • When gas particles absorb thermal energy their kinetic energy increases, i. e. they move more quickly. • The average kinetic energy of the particles of a gas (or any matter) is measured as temperature.
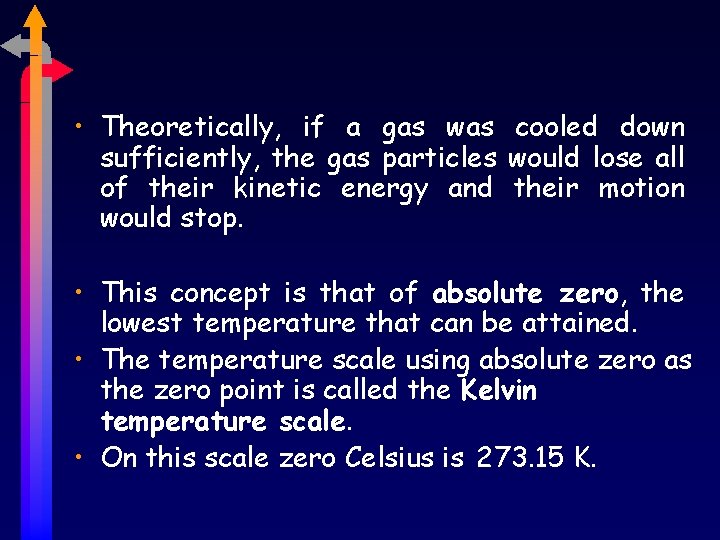
• Theoretically, if a gas was cooled down sufficiently, the gas particles would lose all of their kinetic energy and their motion would stop. • This concept is that of absolute zero, the lowest temperature that can be attained. • The temperature scale using absolute zero as the zero point is called the Kelvin temperature scale. • On this scale zero Celsius is 273. 15 K.
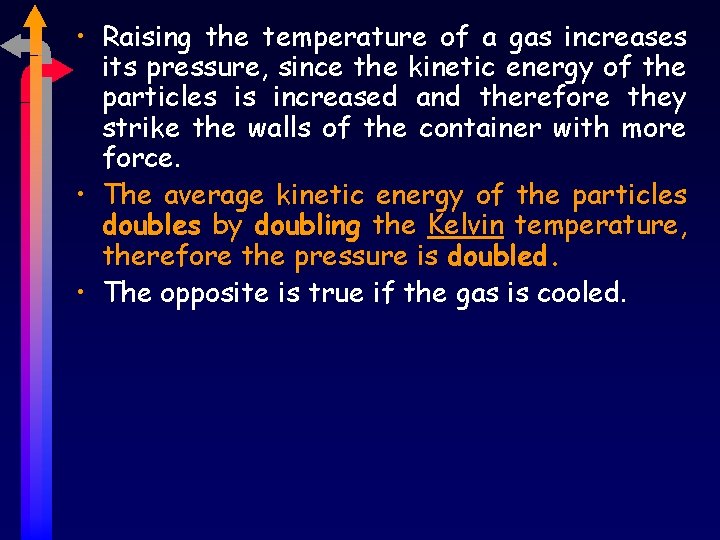
• Raising the temperature of a gas increases its pressure, since the kinetic energy of the particles is increased and therefore they strike the walls of the container with more force. • The average kinetic energy of the particles doubles by doubling the Kelvin temperature, therefore the pressure is doubled. • The opposite is true if the gas is cooled.
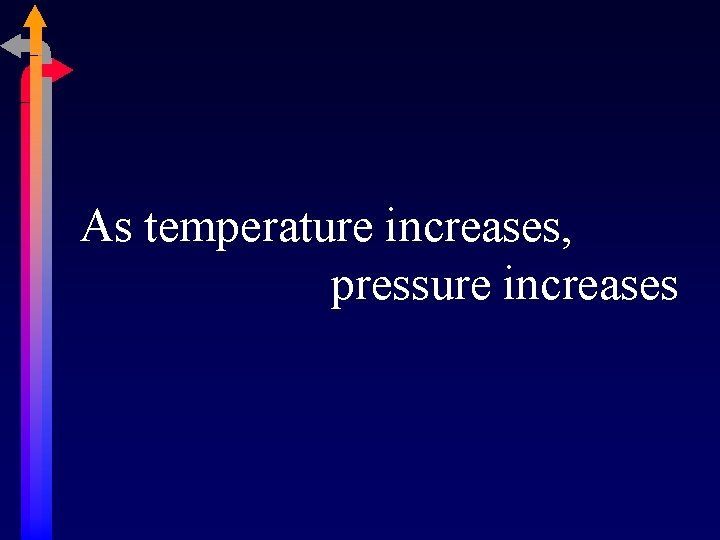
As temperature increases, pressure increases
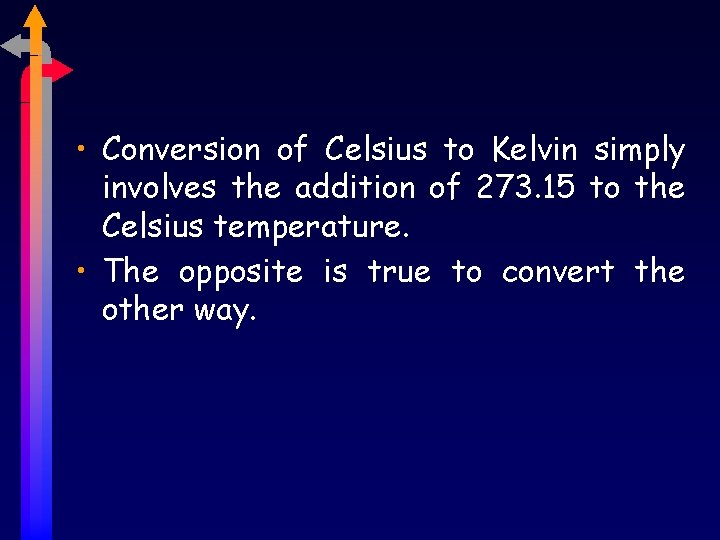
• Conversion of Celsius to Kelvin simply involves the addition of 273. 15 to the Celsius temperature. • The opposite is true to convert the other way.
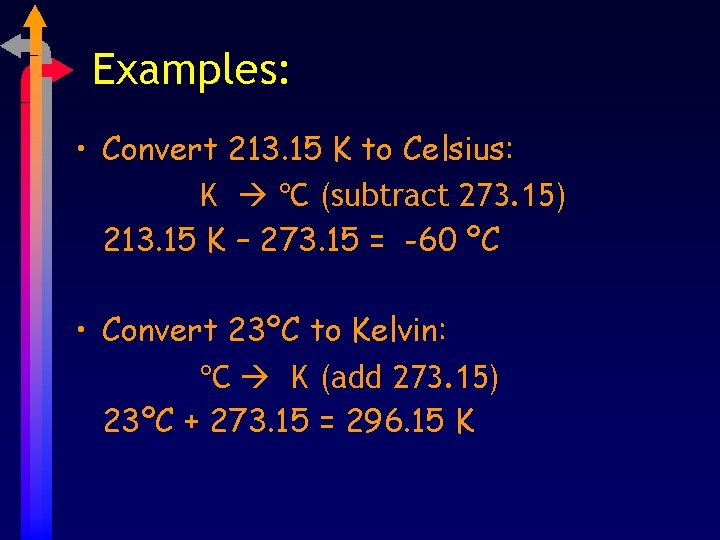
Examples: • Convert 213. 15 K to Celsius: K ºC (subtract 273. 15) 213. 15 K – 273. 15 = -60 ºC • Convert 23ºC to Kelvin: ºC K (add 273. 15) 23ºC + 273. 15 = 296. 15 K
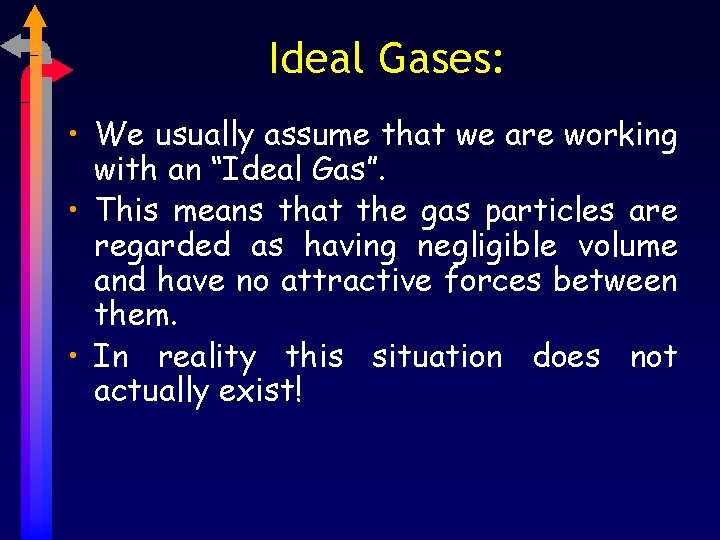
Ideal Gases: • We usually assume that we are working with an “Ideal Gas”. • This means that the gas particles are regarded as having negligible volume and have no attractive forces between them. • In reality this situation does not actually exist!
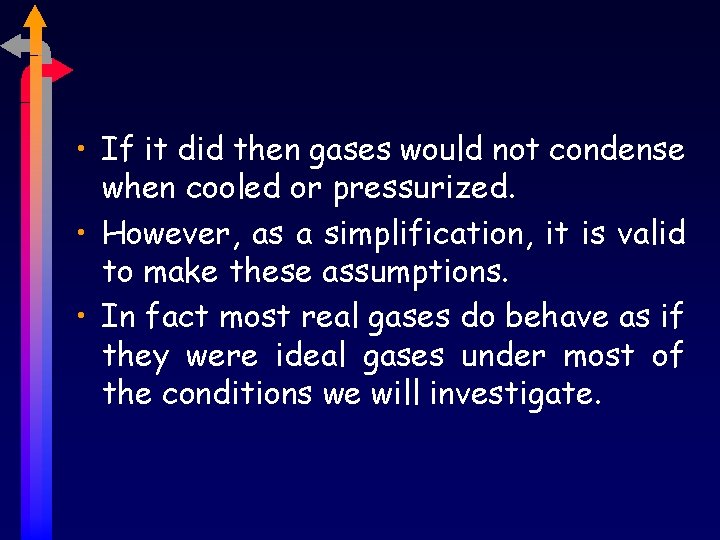
• If it did then gases would not condense when cooled or pressurized. • However, as a simplification, it is valid to make these assumptions. • In fact most real gases do behave as if they were ideal gases under most of the conditions we will investigate.
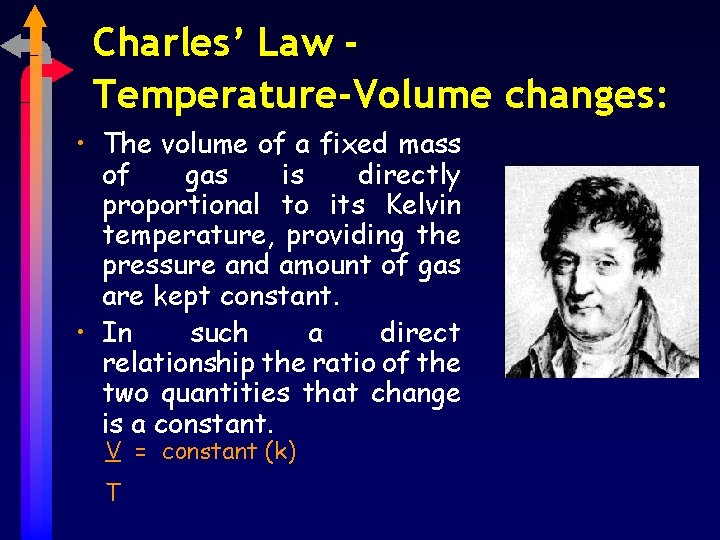
Charles’ Law Temperature-Volume changes: • The volume of a fixed mass of gas is directly proportional to its Kelvin temperature, providing the pressure and amount of gas are kept constant. • In such a direct relationship the ratio of the two quantities that change is a constant. V = constant (k) T
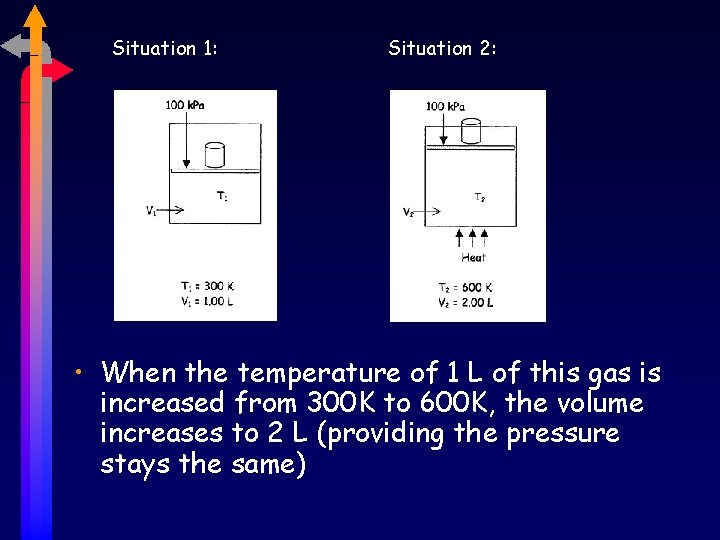
Situation 1: Situation 2: • When the temperature of 1 L of this gas is increased from 300 K to 600 K, the volume increases to 2 L (providing the pressure stays the same)
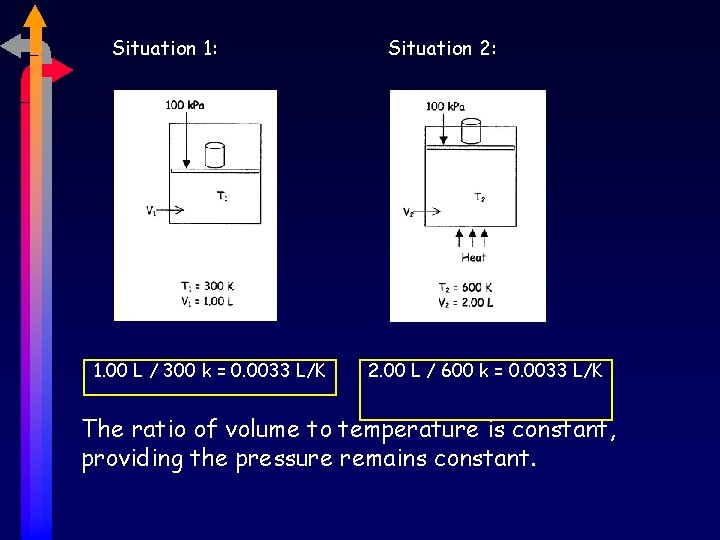
Situation 1: 1. 00 L / 300 k = 0. 0033 L/K Situation 2: 2. 00 L / 600 k = 0. 0033 L/K The ratio of volume to temperature is constant, providing the pressure remains constant.
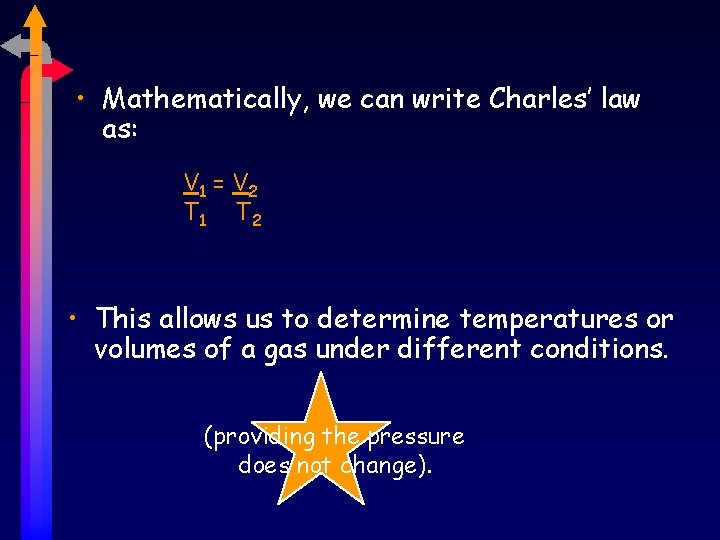
• Mathematically, we can write Charles’ law as: V 1 = V 2 T 1 T 2 • This allows us to determine temperatures or volumes of a gas under different conditions. (providing the pressure does not change).
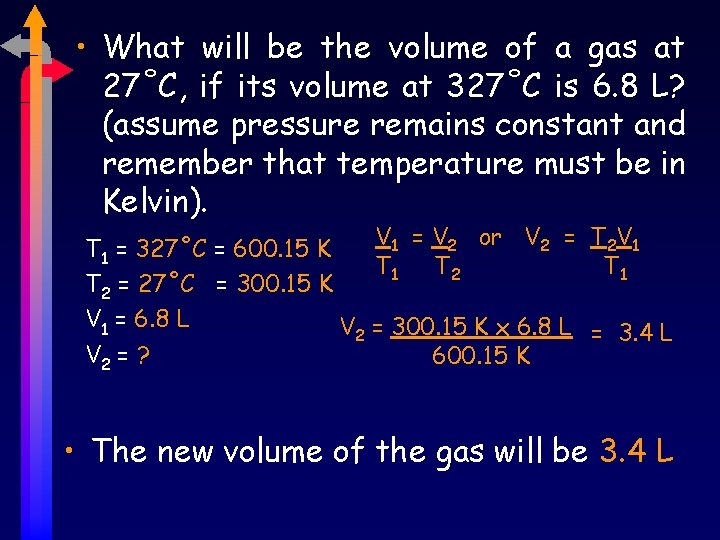
• What will be the volume of a gas at 27˚C, if its volume at 327˚C is 6. 8 L? (assume pressure remains constant and remember that temperature must be in Kelvin). V 1 = V 2 or V 2 = T 2 V 1 T 1 = 327˚C = 600. 15 K T 1 T 2 = 27˚C = 300. 15 K V 1 = 6. 8 L V 2 = 300. 15 K x 6. 8 L = 3. 4 L V 2 = ? 600. 15 K • The new volume of the gas will be 3. 4 L
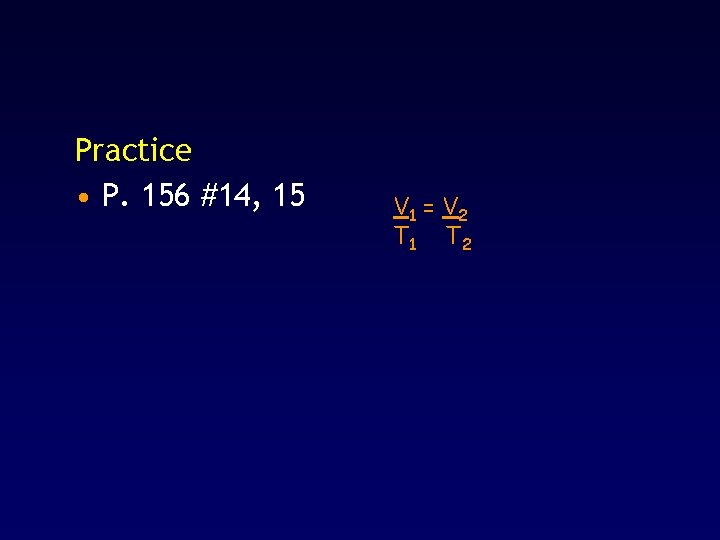
Practice • P. 156 #14, 15 V 1 = V 2 T 1 T 2
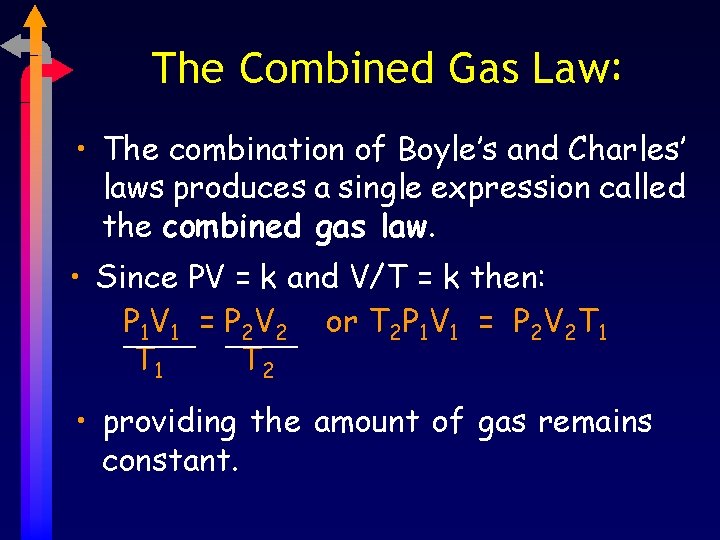
The Combined Gas Law: • The combination of Boyle’s and Charles’ laws produces a single expression called the combined gas law. • Since PV = k and V/T = k then: P 1 V 1 = P 2 V 2 or T 2 P 1 V 1 = P 2 V 2 T 1 T 2 • providing the amount of gas remains constant.
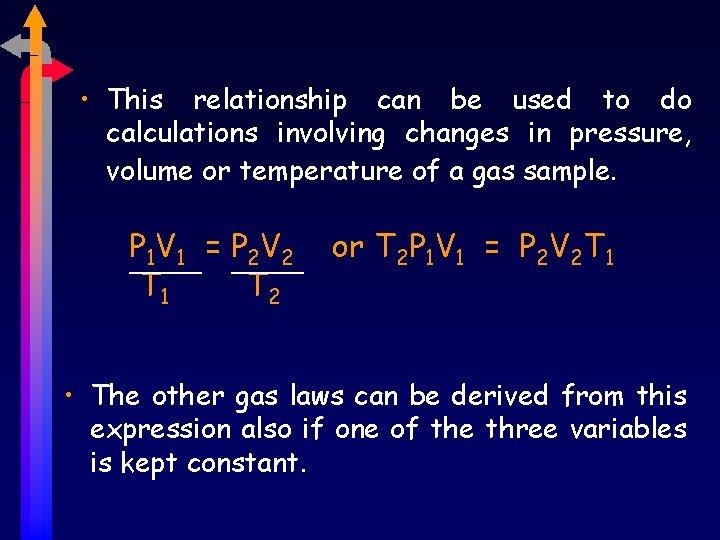
• This relationship can be used to do calculations involving changes in pressure, volume or temperature of a gas sample. P 1 V 1 = P 2 V 2 T 1 T 2 or T 2 P 1 V 1 = P 2 V 2 T 1 • The other gas laws can be derived from this expression also if one of the three variables is kept constant.
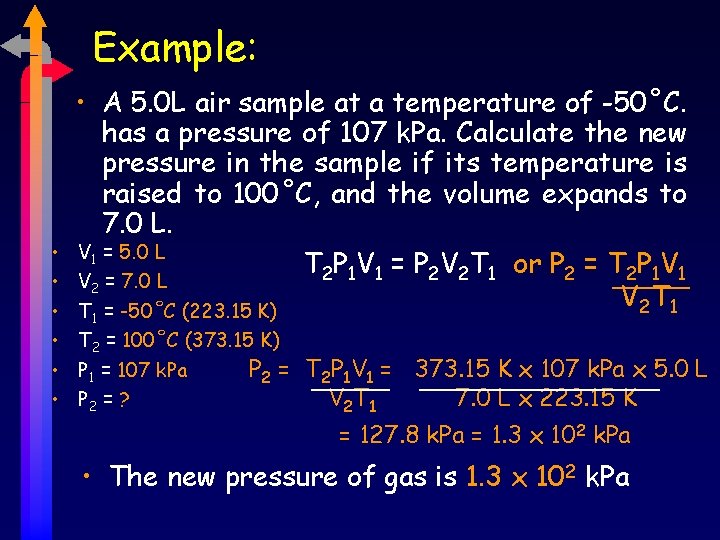
Example: • A 5. 0 L air sample at a temperature of -50˚C. has a pressure of 107 k. Pa. Calculate the new pressure in the sample if its temperature is raised to 100˚C, and the volume expands to 7. 0 L. V 1 = 5. 0 L T 2 P 1 V 1 = P 2 V 2 T 1 or P 2 = T 2 P 1 V 1 V 2 = 7. 0 L V 2 T 1 T = -50˚C (223. 15 K) • • • 1 • T 2 = 100˚C (373. 15 K) P 2 = T 2 P 1 V 1 = • P 1 = 107 k. Pa V 2 T 1 • P 2 = ? 373. 15 K x 107 k. Pa x 5. 0 L 7. 0 L x 223. 15 K = 127. 8 k. Pa = 1. 3 x 102 k. Pa • The new pressure of gas is 1. 3 x 102 k. Pa
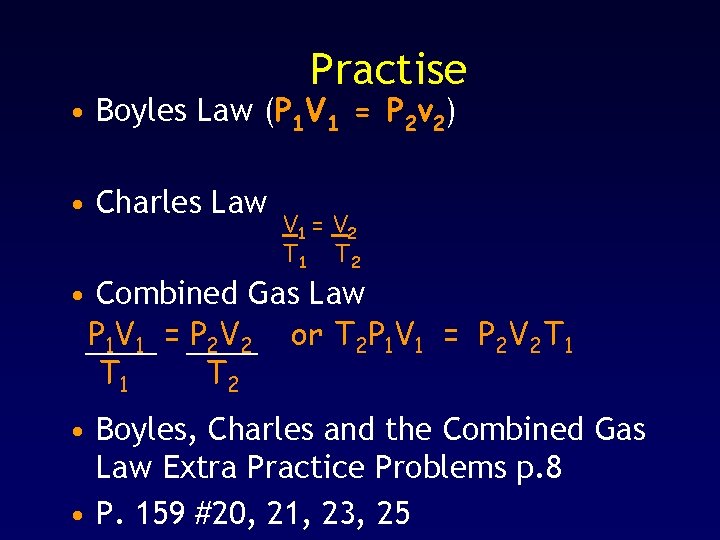
Practise • Boyles Law (P 1 V 1 = P 2 v 2) • Charles Law V 1 = V 2 T 1 T 2 • Combined Gas Law P 1 V 1 = P 2 V 2 or T 2 P 1 V 1 = P 2 V 2 T 1 T 2 • Boyles, Charles and the Combined Gas Law Extra Practice Problems p. 8 • P. 159 #20, 21, 23, 25
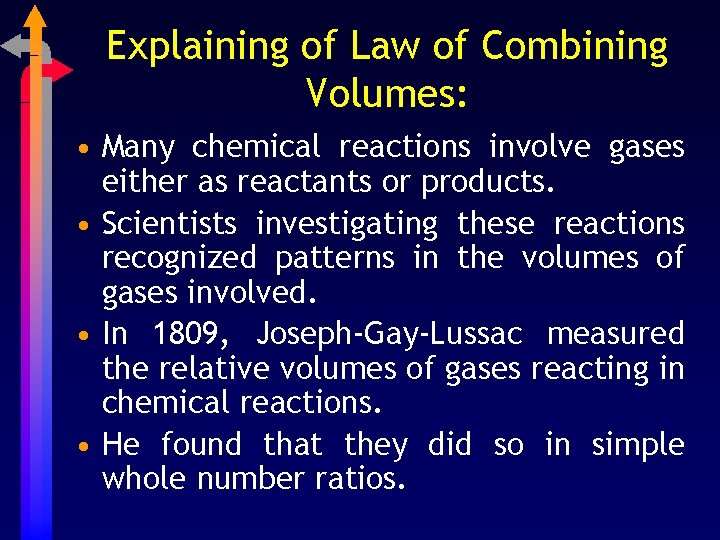
Explaining of Law of Combining Volumes: • Many chemical reactions involve gases either as reactants or products. • Scientists investigating these reactions recognized patterns in the volumes of gases involved. • In 1809, Joseph-Gay-Lussac measured the relative volumes of gases reacting in chemical reactions. • He found that they did so in simple whole number ratios.
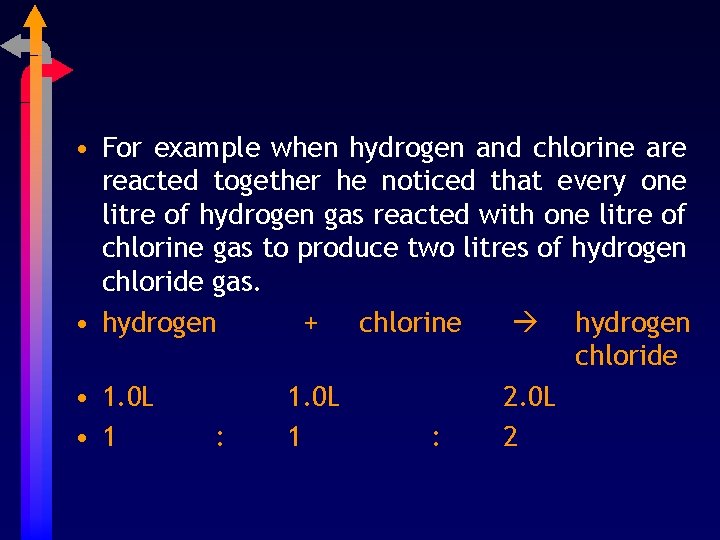
• For example when hydrogen and chlorine are reacted together he noticed that every one litre of hydrogen gas reacted with one litre of chlorine gas to produce two litres of hydrogen chloride gas. • hydrogen + chlorine hydrogen chloride • 1. 0 L 2. 0 L • 1 : 2
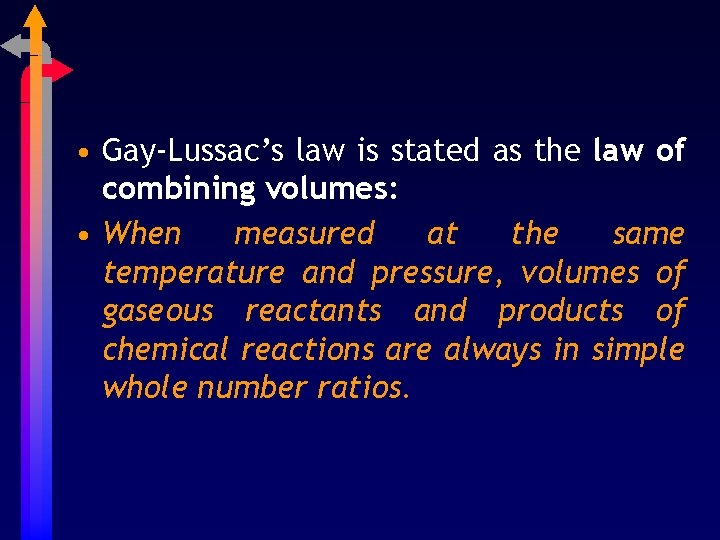
• Gay-Lussac’s law is stated as the law of combining volumes: • When measured at the same temperature and pressure, volumes of gaseous reactants and products of chemical reactions are always in simple whole number ratios.
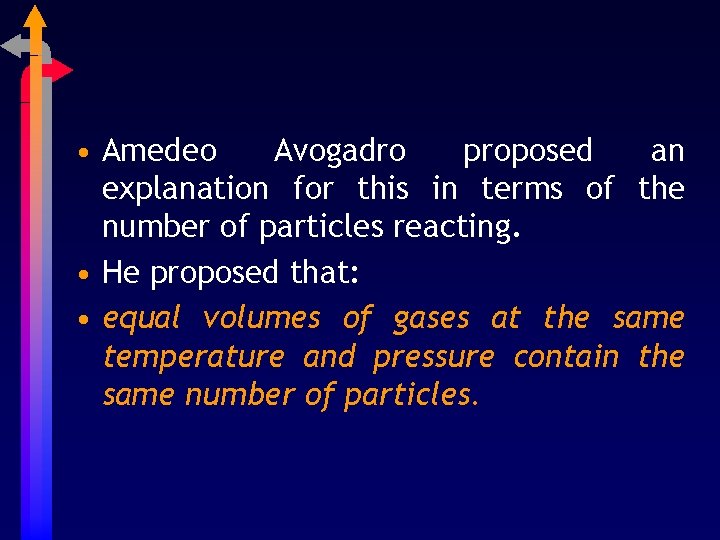
• Amedeo Avogadro proposed an explanation for this in terms of the number of particles reacting. • He proposed that: • equal volumes of gases at the same temperature and pressure contain the same number of particles.
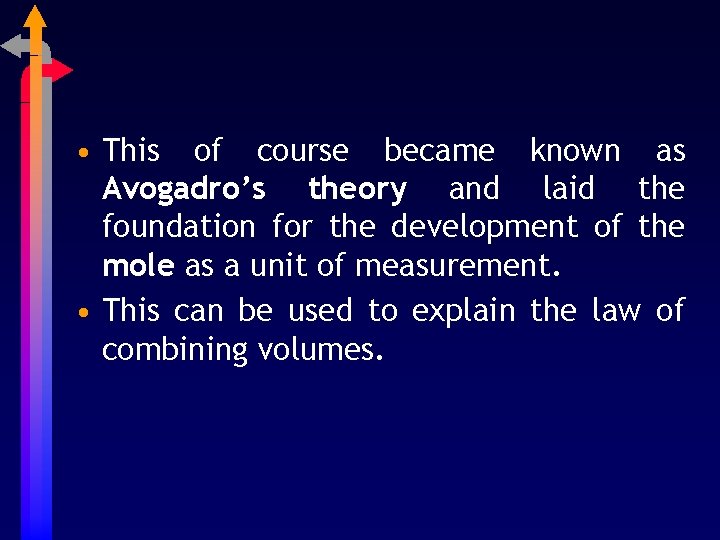
• This of course became known as Avogadro’s theory and laid the foundation for the development of the mole as a unit of measurement. • This can be used to explain the law of combining volumes.
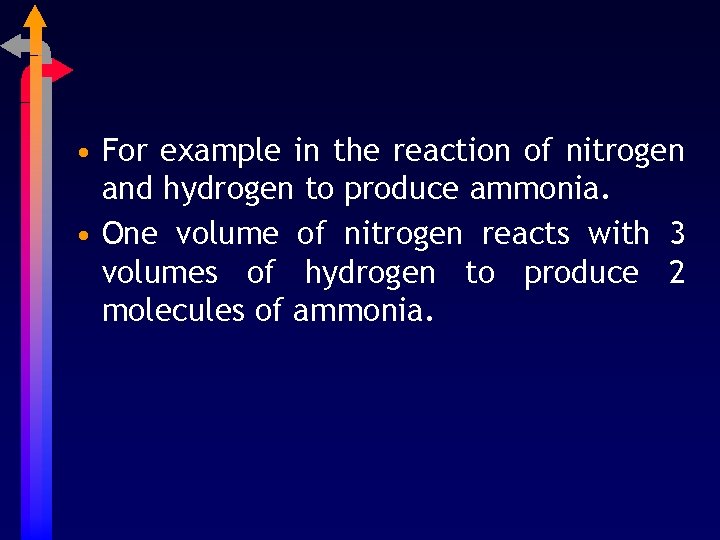
• For example in the reaction of nitrogen and hydrogen to produce ammonia. • One volume of nitrogen reacts with 3 volumes of hydrogen to produce 2 molecules of ammonia.
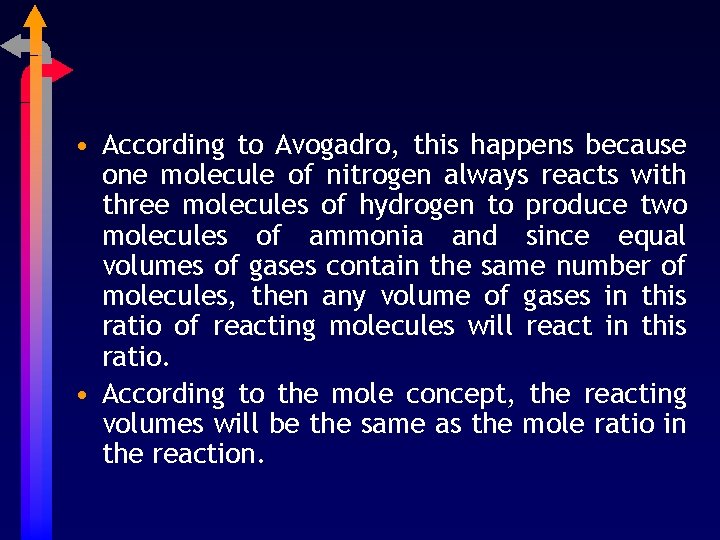
• According to Avogadro, this happens because one molecule of nitrogen always reacts with three molecules of hydrogen to produce two molecules of ammonia and since equal volumes of gases contain the same number of molecules, then any volume of gases in this ratio of reacting molecules will react in this ratio. • According to the mole concept, the reacting volumes will be the same as the mole ratio in the reaction.
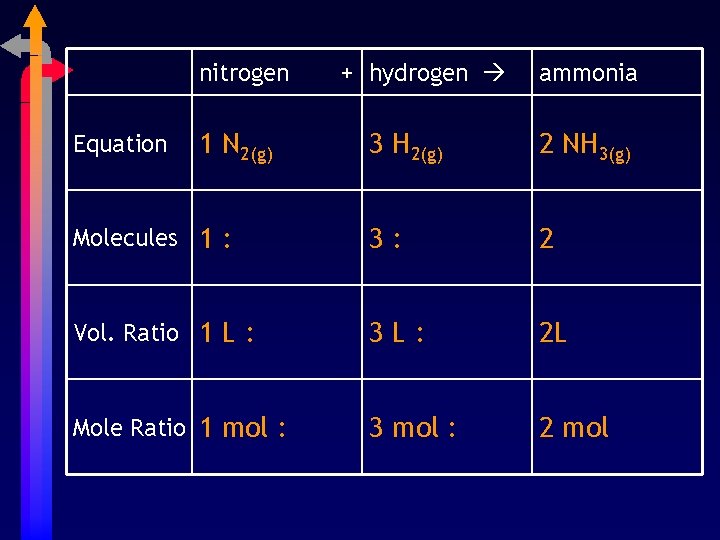
nitrogen ammonia 3 H 2(g) 2 NH 3(g) Molecules 1 : 3: 2 Vol. Ratio 1 L : 3 L: 2 L Mole Ratio 1 mol : 3 mol : 2 mol Equation 1 N 2(g) + hydrogen
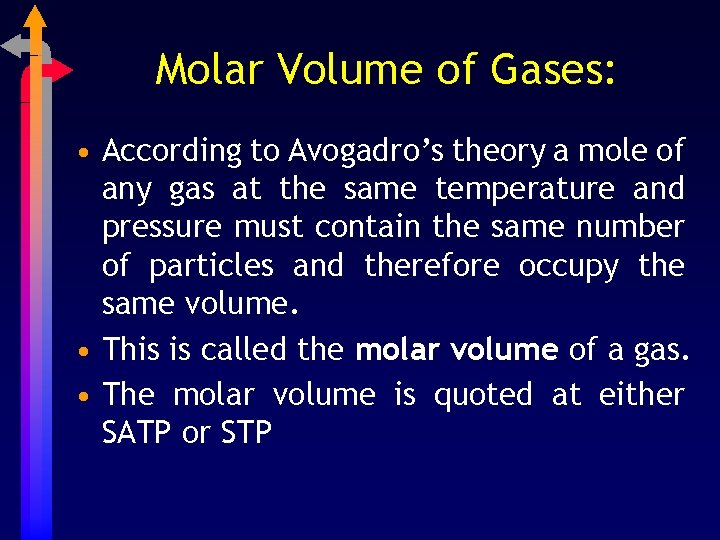
Molar Volume of Gases: • According to Avogadro’s theory a mole of any gas at the same temperature and pressure must contain the same number of particles and therefore occupy the same volume. • This is called the molar volume of a gas. • The molar volume is quoted at either SATP or STP
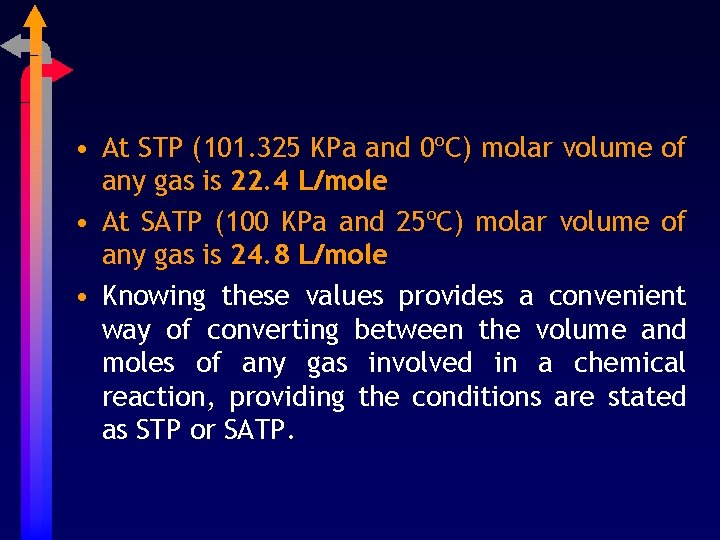
• At STP (101. 325 KPa and 0ºC) molar volume of any gas is 22. 4 L/mole • At SATP (100 KPa and 25ºC) molar volume of any gas is 24. 8 L/mole • Knowing these values provides a convenient way of converting between the volume and moles of any gas involved in a chemical reaction, providing the conditions are stated as STP or SATP.
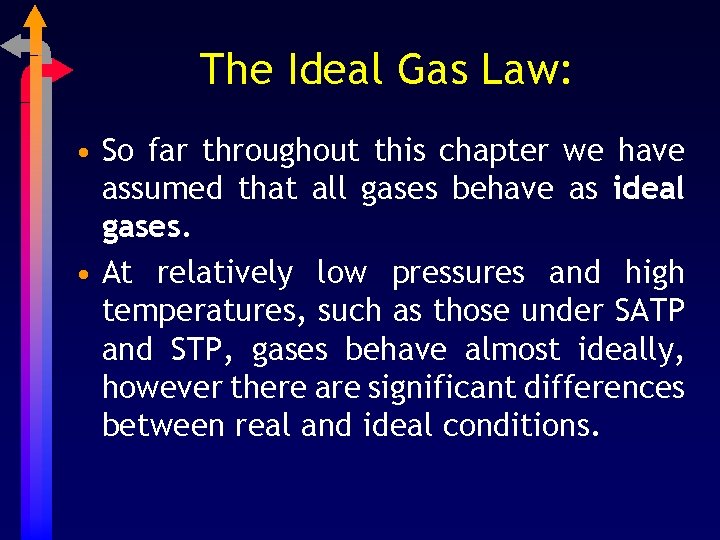
The Ideal Gas Law: • So far throughout this chapter we have assumed that all gases behave as ideal gases. • At relatively low pressures and high temperatures, such as those under SATP and STP, gases behave almost ideally, however there are significant differences between real and ideal conditions.
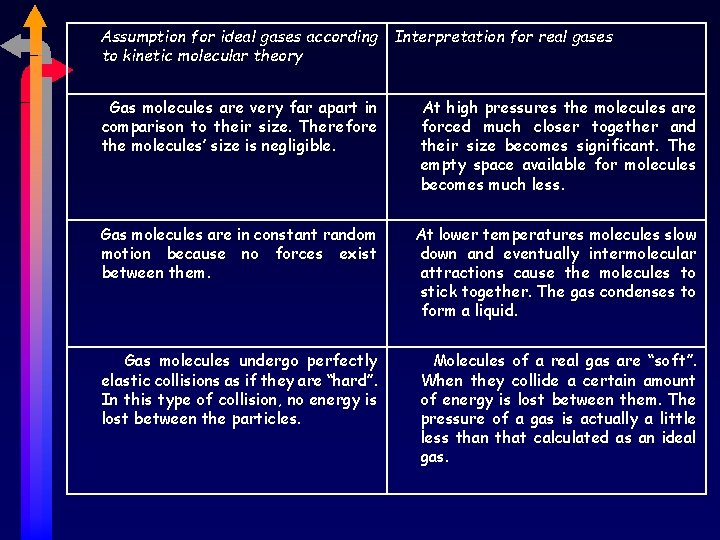
Assumption for ideal gases according Interpretation for real gases to kinetic molecular theory Gas molecules are very far apart in comparison to their size. Therefore the molecules’ size is negligible. At high pressures the molecules are forced much closer together and their size becomes significant. The empty space available for molecules becomes much less. Gas molecules are in constant random motion because no forces exist between them. At lower temperatures molecules slow down and eventually intermolecular attractions cause the molecules to stick together. The gas condenses to form a liquid. Gas molecules undergo perfectly elastic collisions as if they are “hard”. In this type of collision, no energy is lost between the particles. Molecules of a real gas are “soft”. When they collide a certain amount of energy is lost between them. The pressure of a gas is actually a little less than that calculated as an ideal gas.
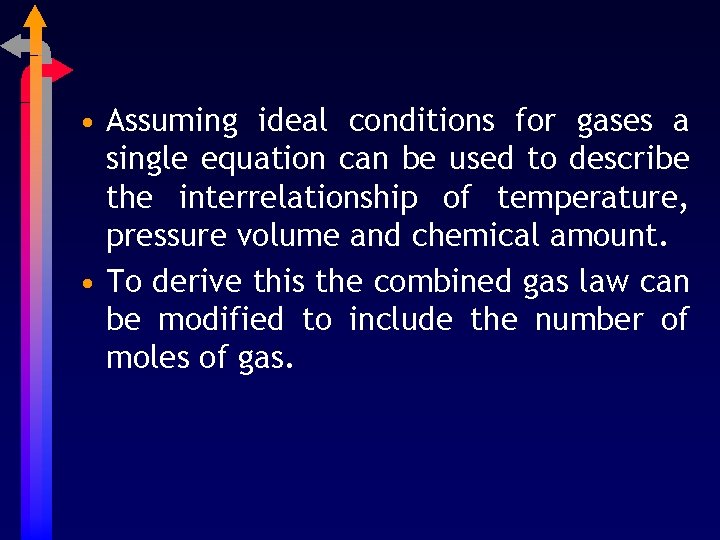
• Assuming ideal conditions for gases a single equation can be used to describe the interrelationship of temperature, pressure volume and chemical amount. • To derive this the combined gas law can be modified to include the number of moles of gas.
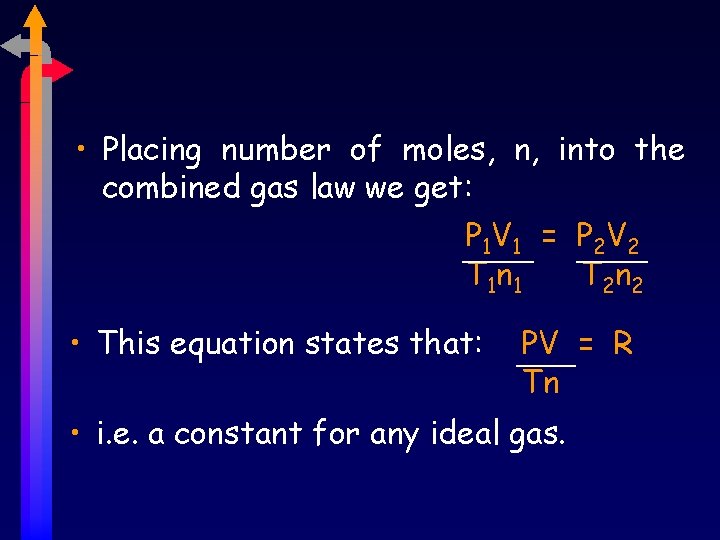
• Placing number of moles, n, into the combined gas law we get: P 1 V 1 = P 2 V 2 T 1 n 1 T 2 n 2 • This equation states that: PV = R Tn • i. e. a constant for any ideal gas.
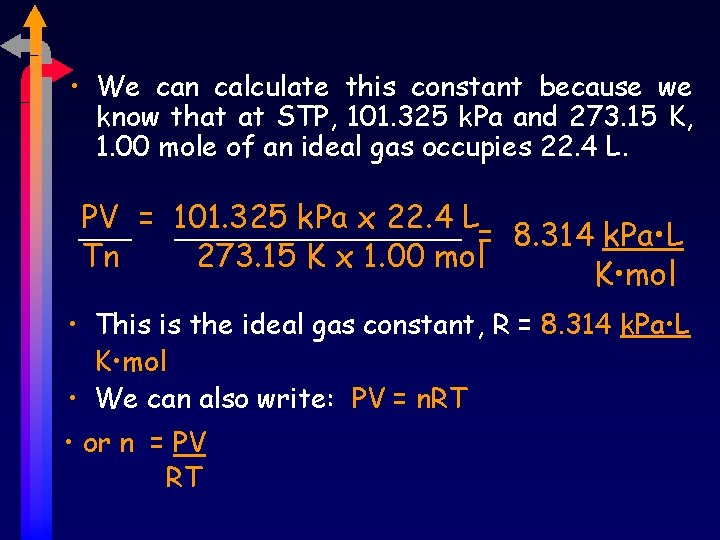
• We can calculate this constant because we know that at STP, 101. 325 k. Pa and 273. 15 K, 1. 00 mole of an ideal gas occupies 22. 4 L. PV = 101. 325 k. Pa x 22. 4 L = 8. 314 k. Pa • L Tn 273. 15 K x 1. 00 mol K • mol • This is the ideal gas constant, R = 8. 314 k. Pa • L K • mol • We can also write: PV = n. RT • or n = PV RT
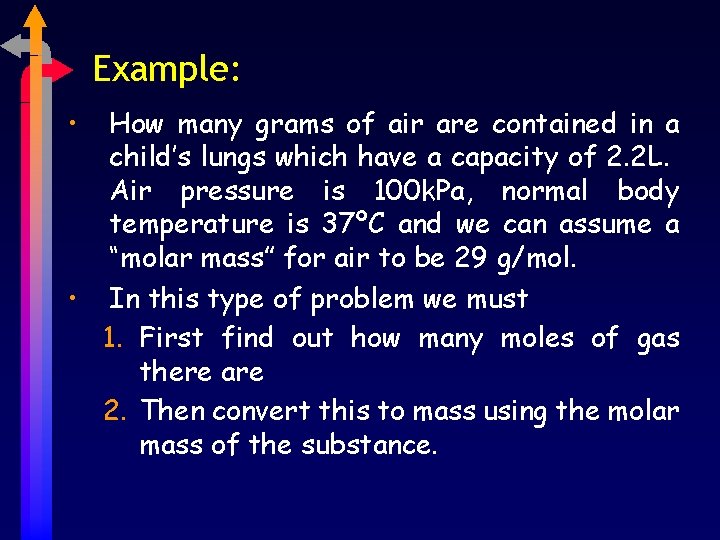
Example: • How many grams of air are contained in a child’s lungs which have a capacity of 2. 2 L. Air pressure is 100 k. Pa, normal body temperature is 37ºC and we can assume a “molar mass” for air to be 29 g/mol. • In this type of problem we must 1. First find out how many moles of gas there are 2. Then convert this to mass using the molar mass of the substance.
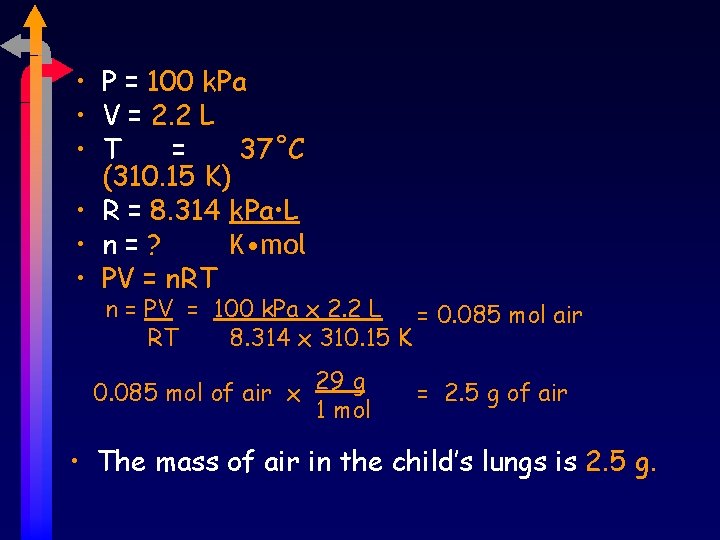
• P = 100 k. Pa • V = 2. 2 L • T = 37˚C (310. 15 K) • R = 8. 314 k. Pa • L • n=? K • mol • PV = n. RT n = PV = 100 k. Pa x 2. 2 L = 0. 085 mol air RT 8. 314 x 310. 15 K 0. 085 mol of air x 29 g 1 mol = 2. 5 g of air • The mass of air in the child’s lungs is 2. 5 g.
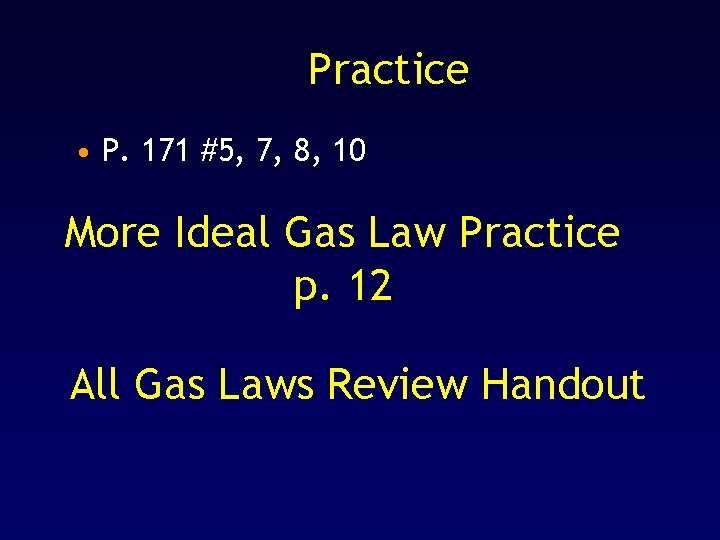
Practice • P. 171 #5, 7, 8, 10 More Ideal Gas Law Practice p. 12 All Gas Laws Review Handout
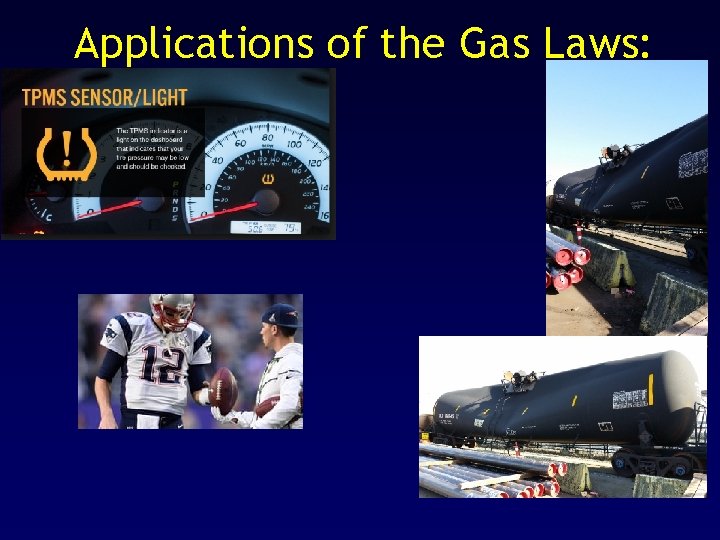
Applications of the Gas Laws:
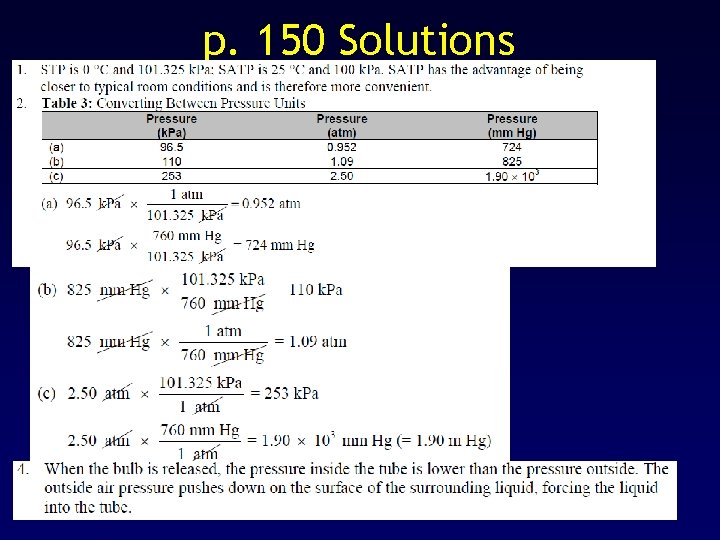
p. 150 Solutions
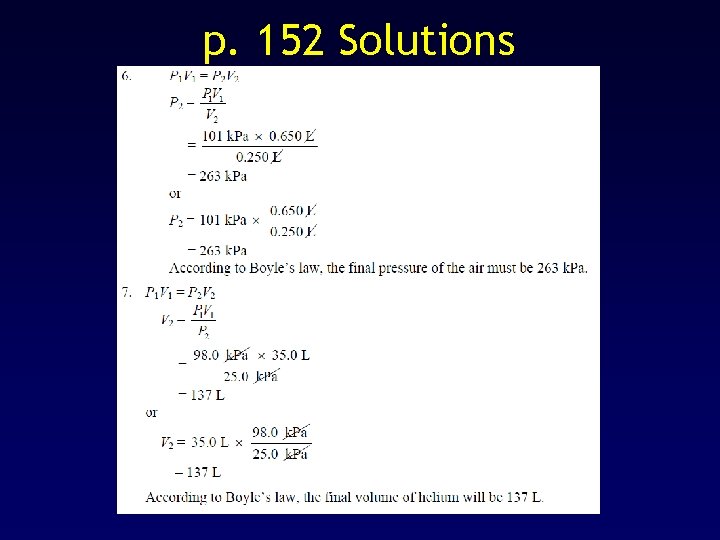
p. 152 Solutions
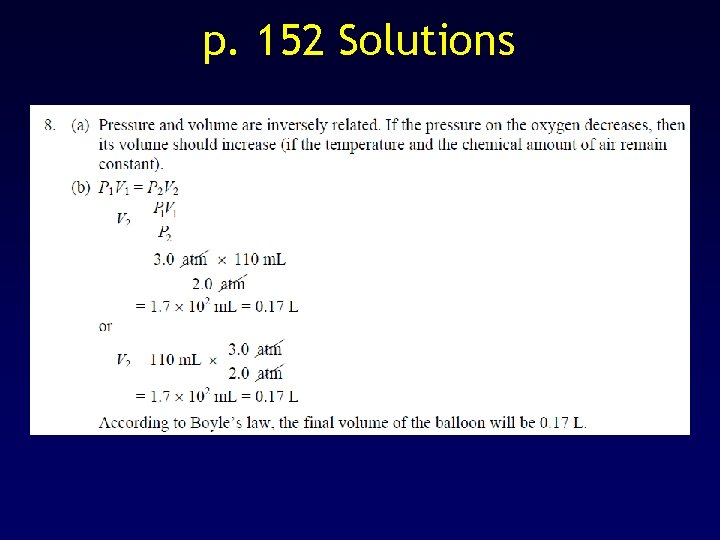
p. 152 Solutions
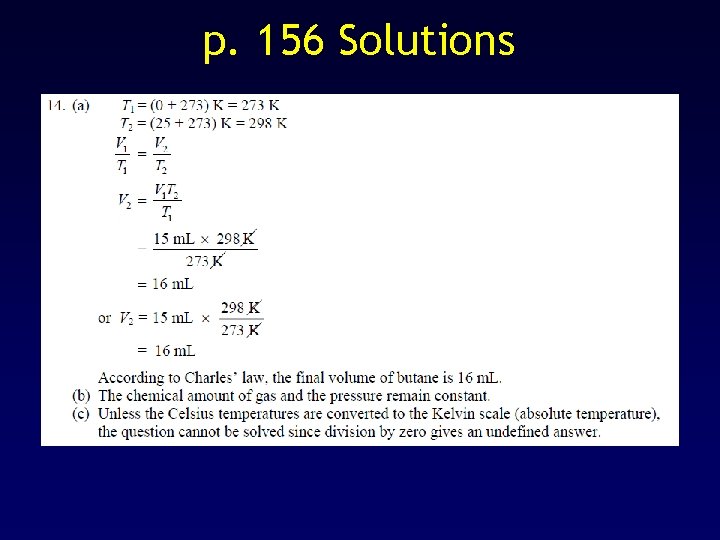
p. 156 Solutions
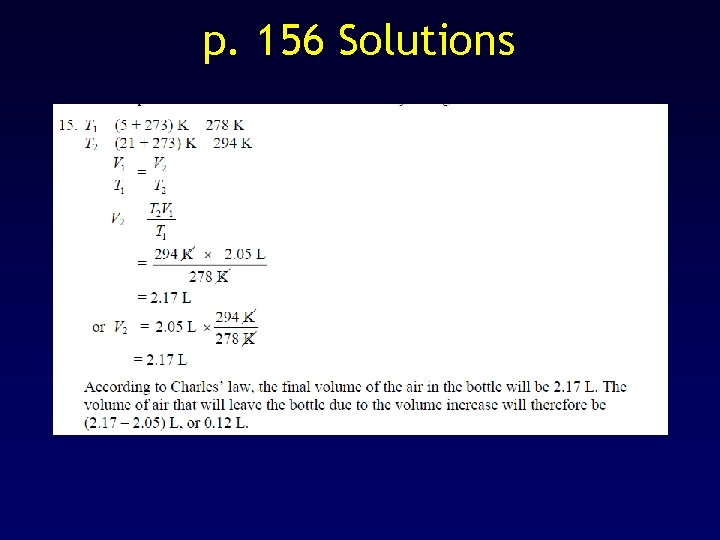
p. 156 Solutions
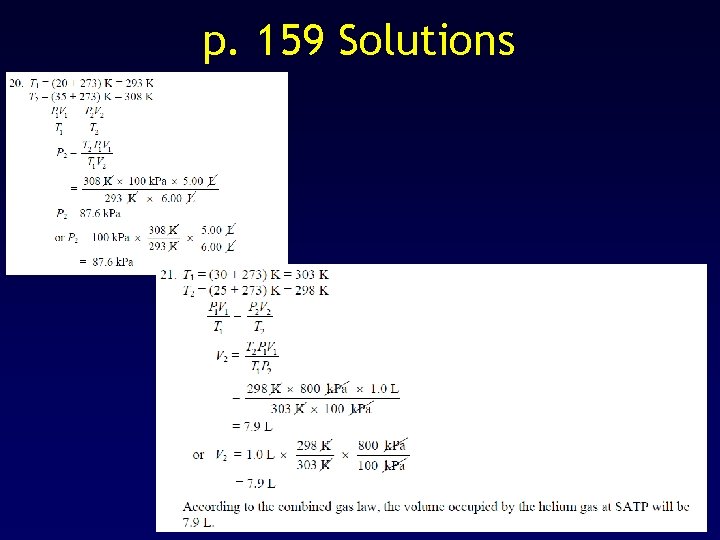
p. 159 Solutions
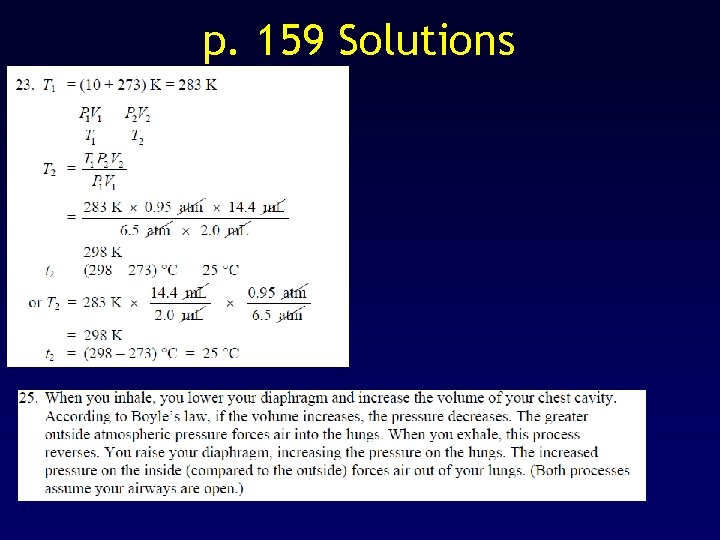
p. 159 Solutions
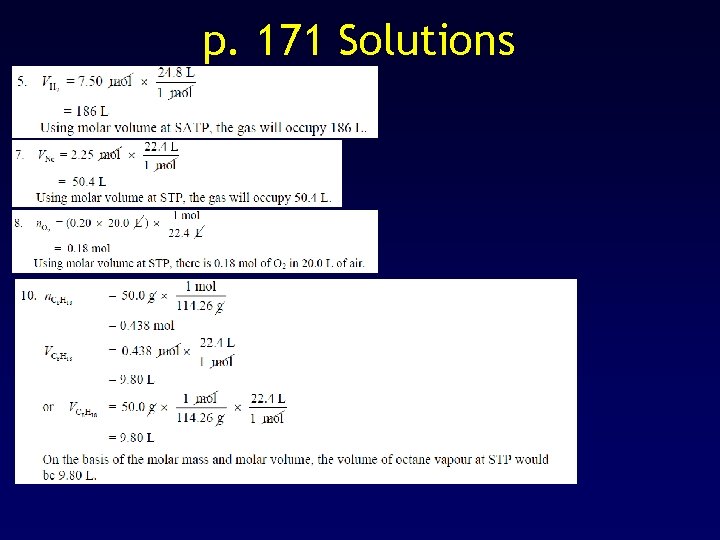
p. 171 Solutions
Chapter 14 solids liquids and gases worksheet answers
Expansion of solids liquids and gases examples
Matter and its composition
Why is gas easier to compress than a liquid or a solid
Chapter 14 solids liquids and gases
Buoyancyability
Motion of particles in solids, liquids and gases
Solid liquid gas examples
Particle movement in solids liquids and gases
Venn diagram of conduction convection and radiation
The science duo physical and chemical changes
How does sound travel through solids liquids and gases
Properties of solid liquid and gas
Filtration
Kinetic molecular theory of liquids and solids
Liquids and solids menu
Kesler science.com
Properties of solid
Chapter 11 - states of matter: liquids and solids
Molecular theory of gases and liquids
Synthesis of ionic liquids ppt
Chemistry chapter 11 gases test
Inorganic chemistry vs organic chemistry
Ib organic chemistry functional groups
Sysco thickened liquids
Plant growth with different liquids
Water is countable or uncountable
Miscible vs immiscible chemistry
Examples of gas
Liquid take the shape of their
Flammable liquids
Type of beaker
Separates liquids of different colours
Chapter 19 liquids exercises answers
"essential liquids inc."
Separation of immiscible liquids diagram
Rocks changed by temperature pressure and hot liquids
14. separating mixtures of liquids.
Why are liquids incompressible
Thickened liquids that complement other foods. *
The monophasic liquid dosage form is solutions.
Thermal expansion in liquids
What liquid freezes the fastest
Nectar thick liquids walmart
Kinetic molecular theory of liquids
Why are liquids incompressible
What are the 3 properties of a liquid
Dynamic viscosity definition
Water the elixir of life essay wikipedia
Concentric tube thief is used for sampling of
Type of fire that involves flammable liquids
Basic baking ingredients
Uncountable nouns liquids
Thermal expansion in liquids
Do all liquids evaporate
Whats a physical change
How dense are you
Viscosity of liquids
Thickening agents for sauce
Thermal expansion in liquids
Frazier free water protocol printable
How many elements are liquids
Liquids phonetics examples
Example of pure liquid dielectric is
Benzene
Electrical conductivity of solid
Example of viscoelastic material
Diffusible and indiffusible suspension
Octahedron vertices
Flowchart undissolved solids
Similar solids
Point defects
The attraction between particles gives solids a definite
Soluble solids definition