Chemical Kinetics Chemical Kinetics Do Now 1 TRUE
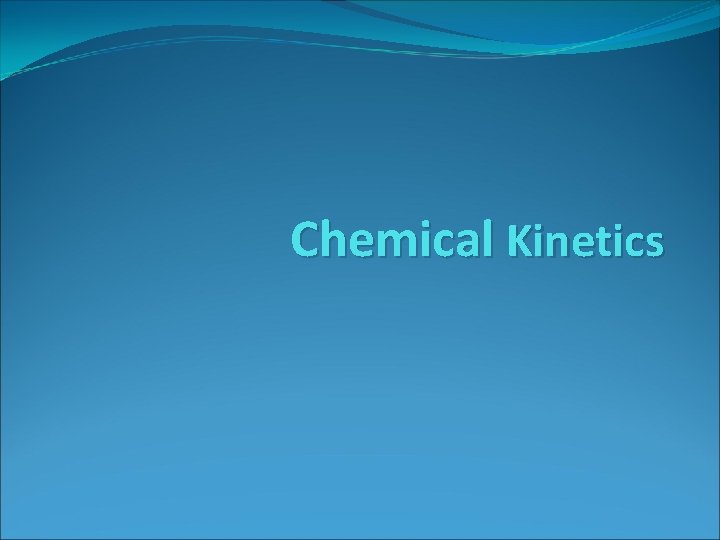
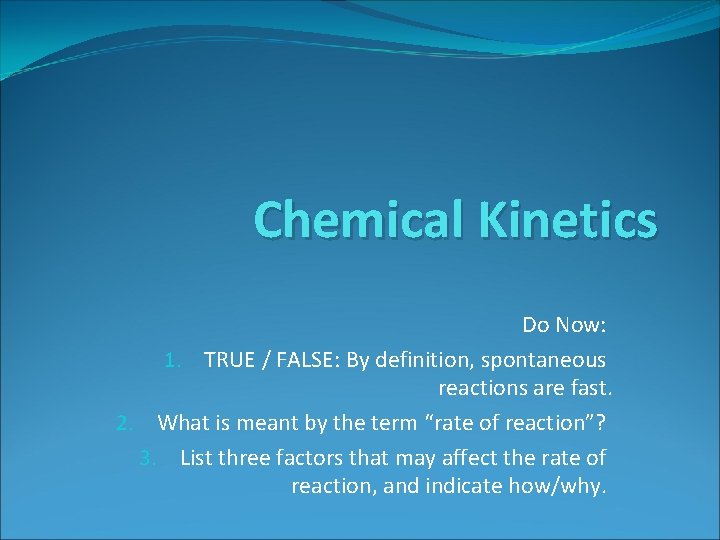
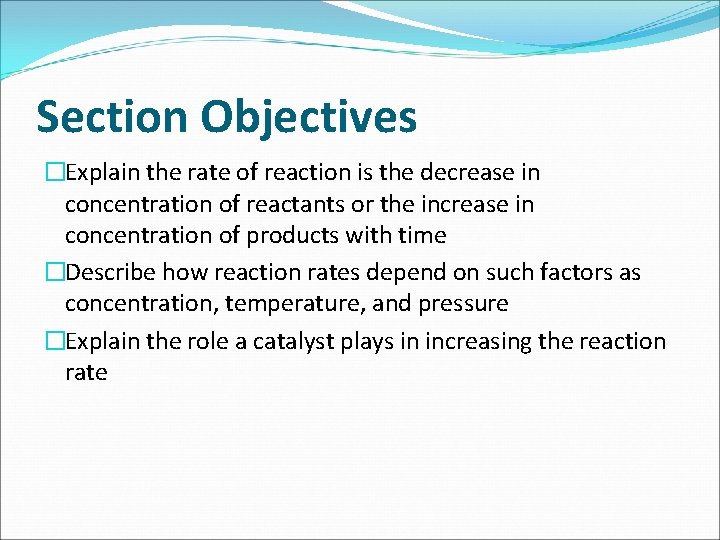
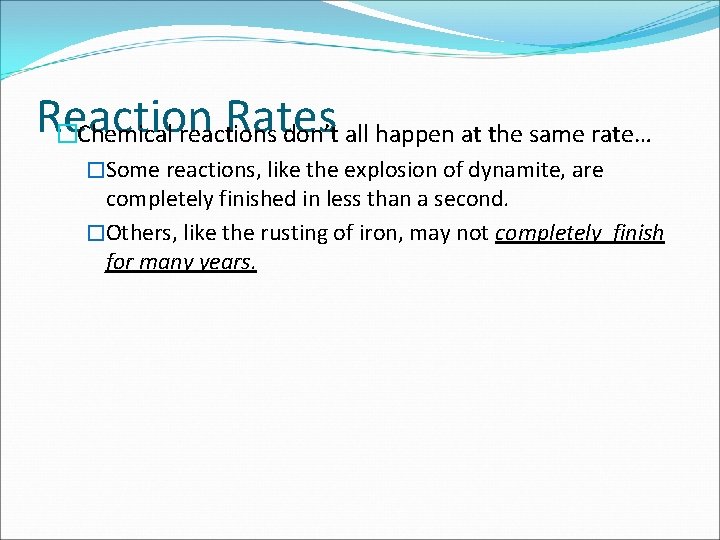
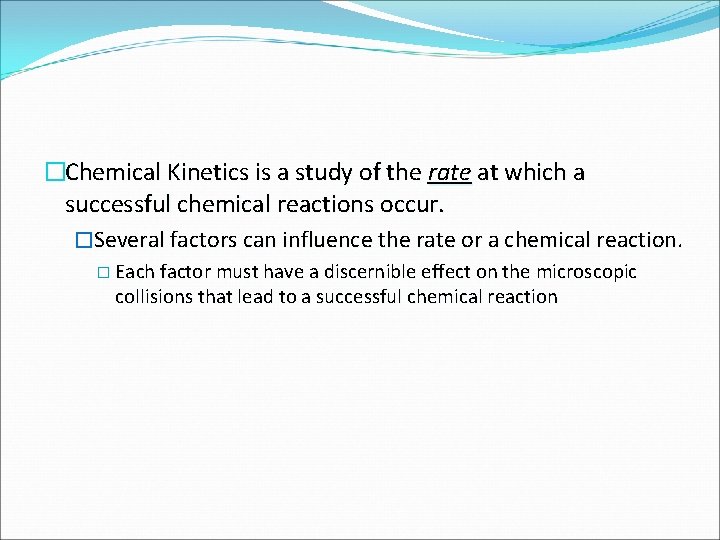
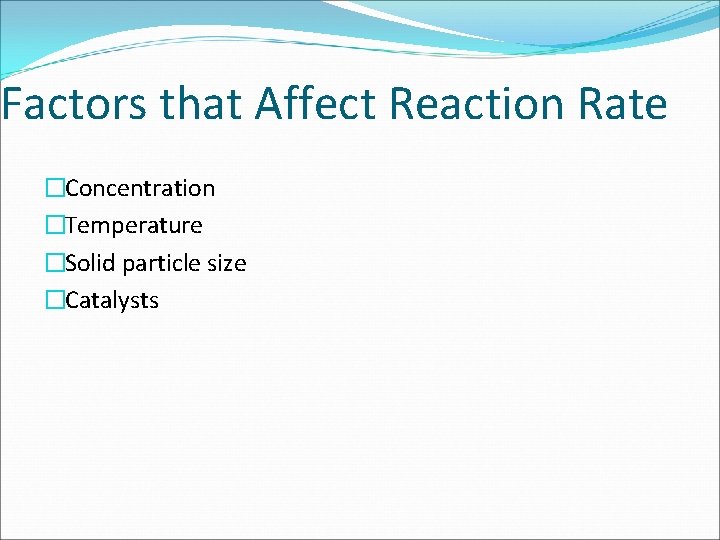
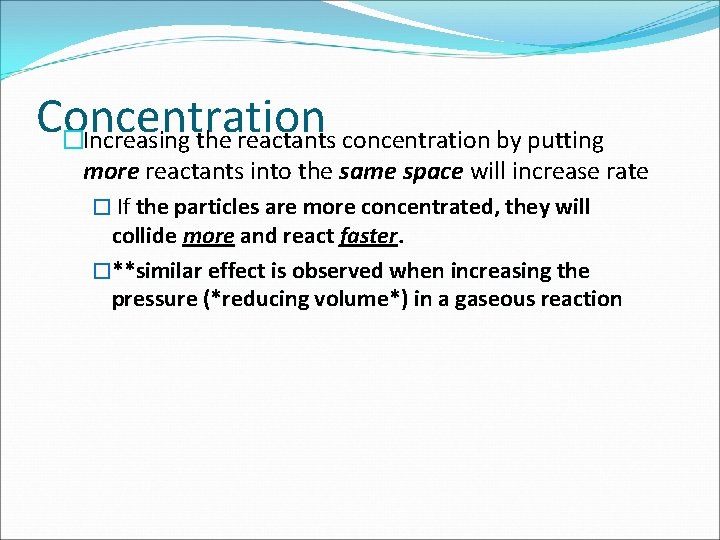
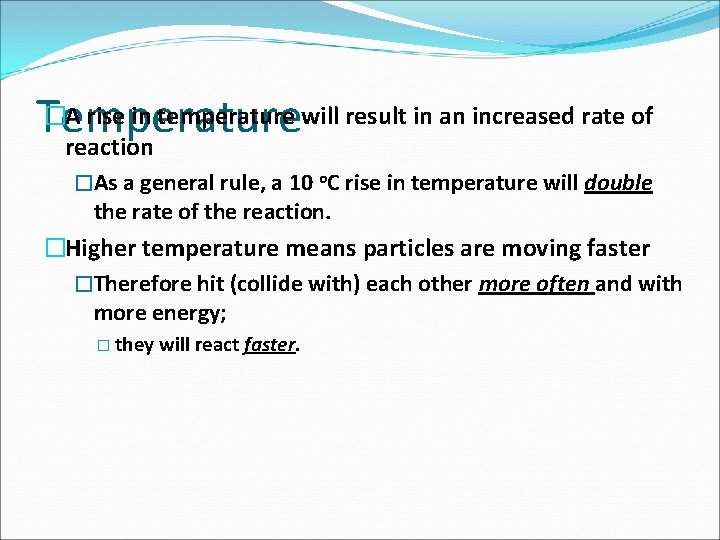
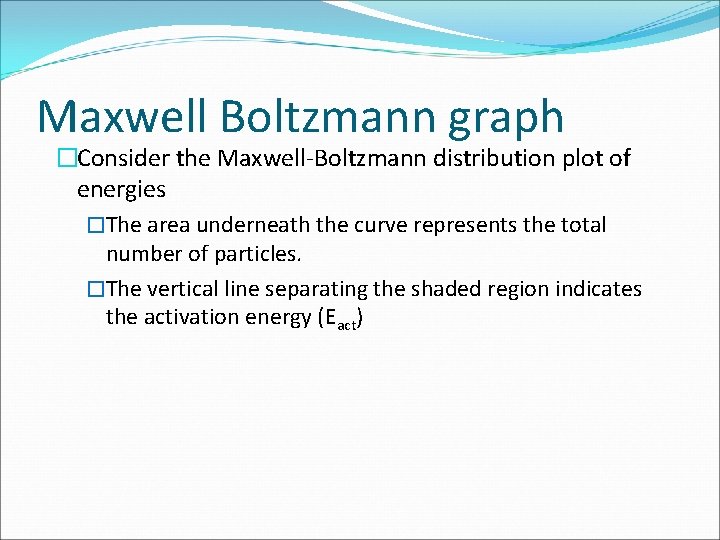
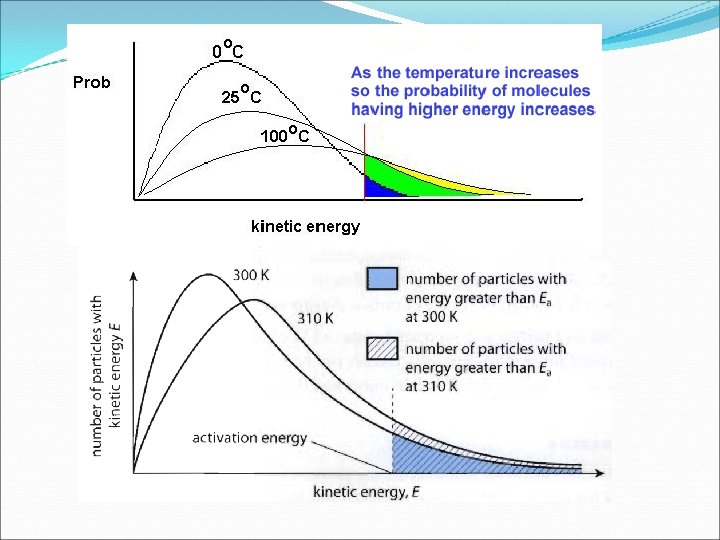
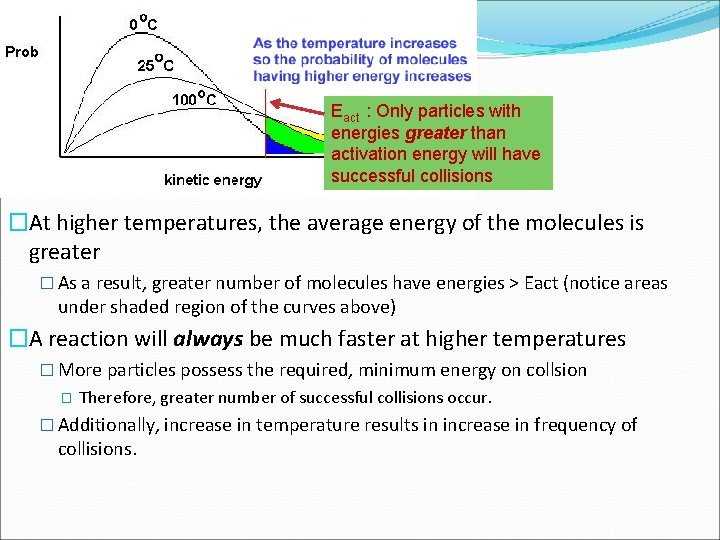
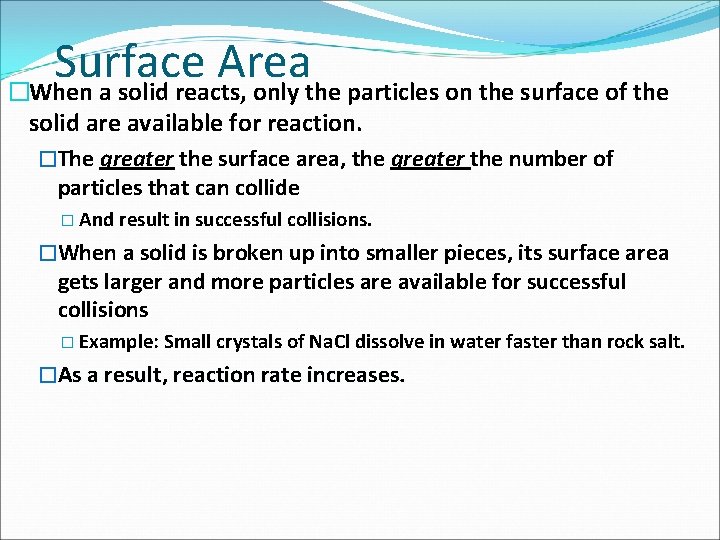
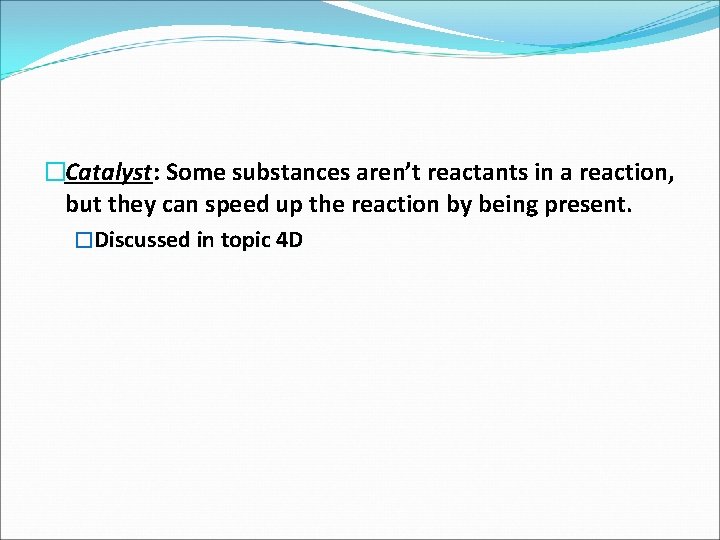
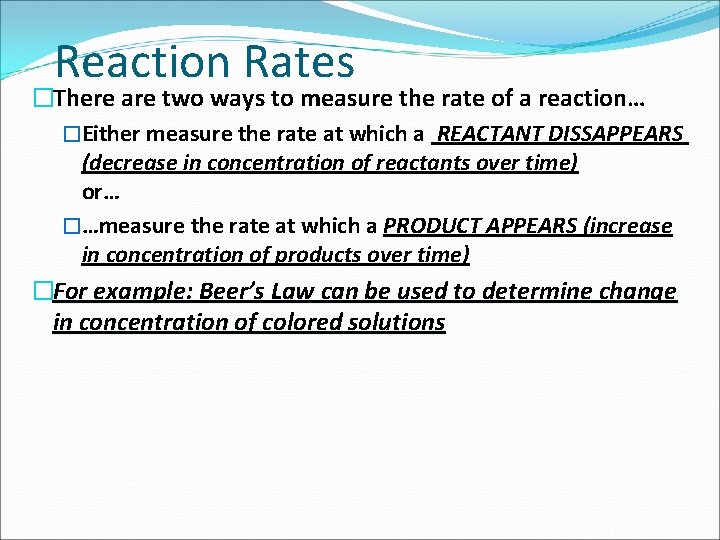
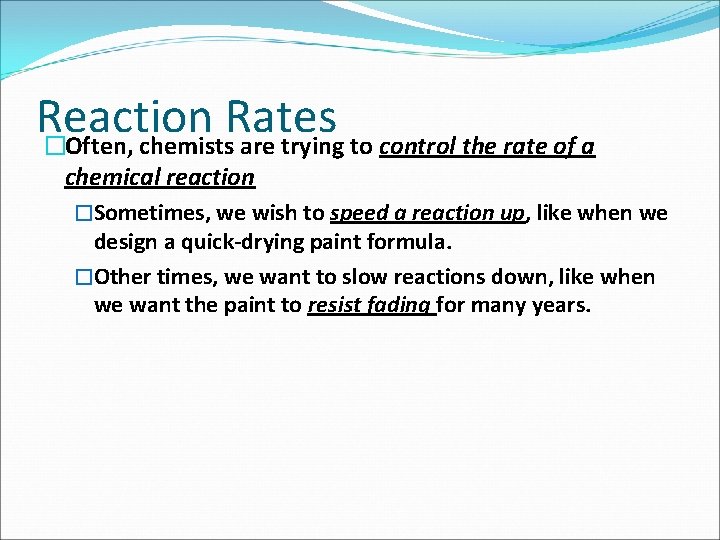
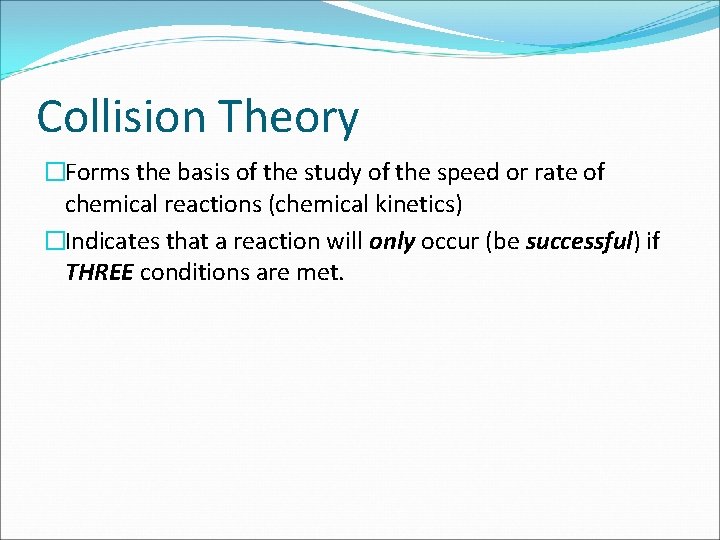
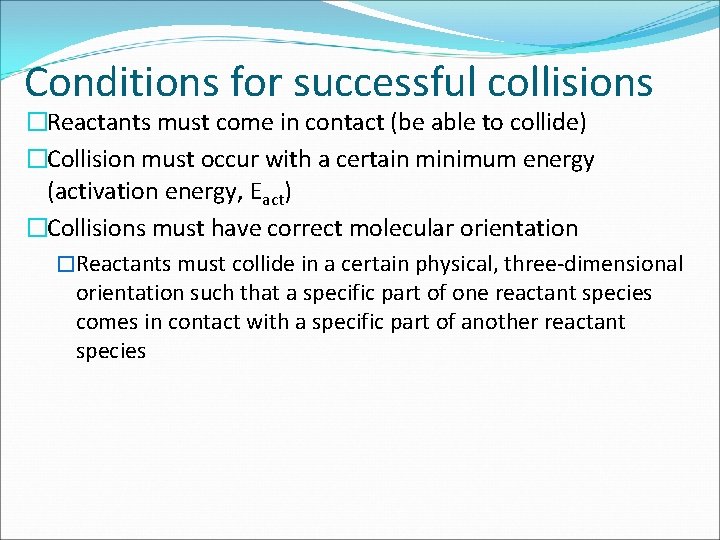
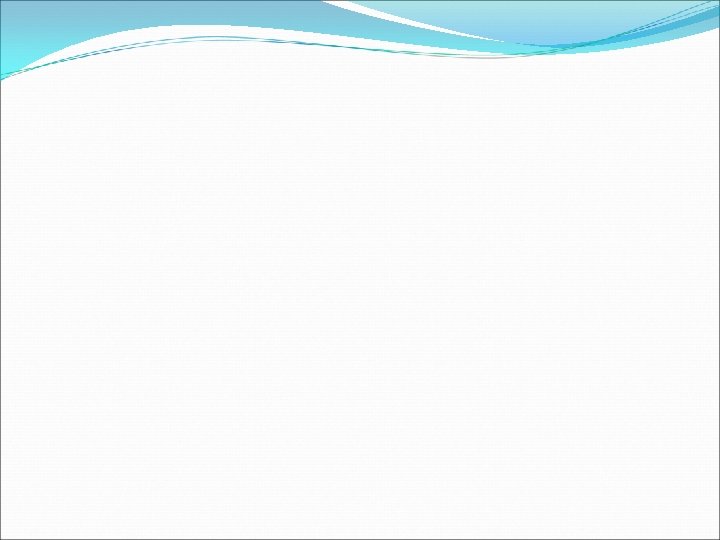
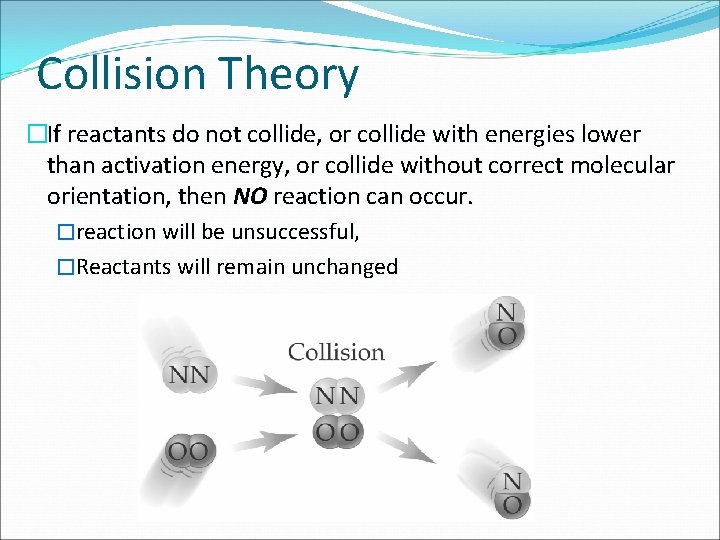
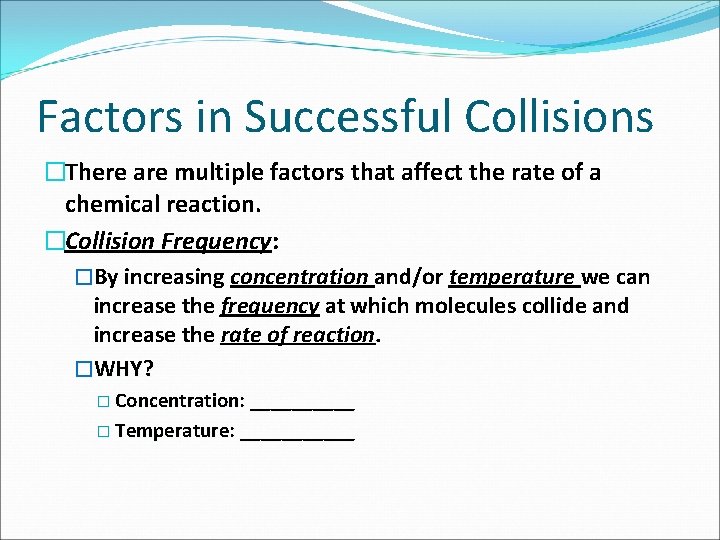
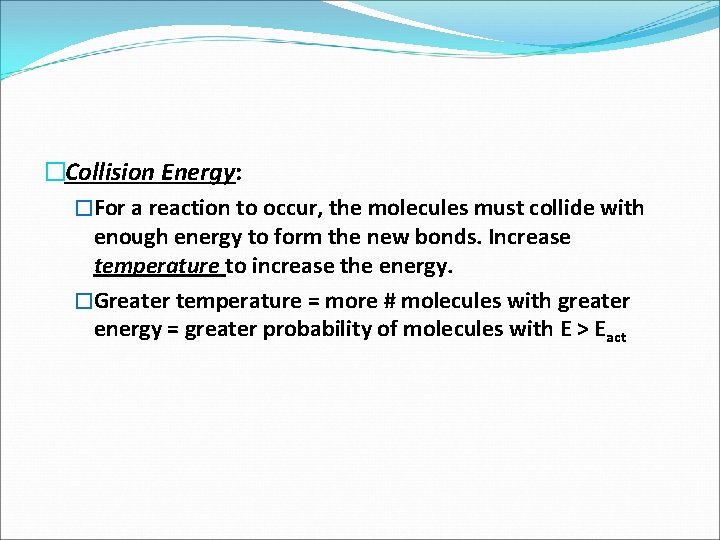
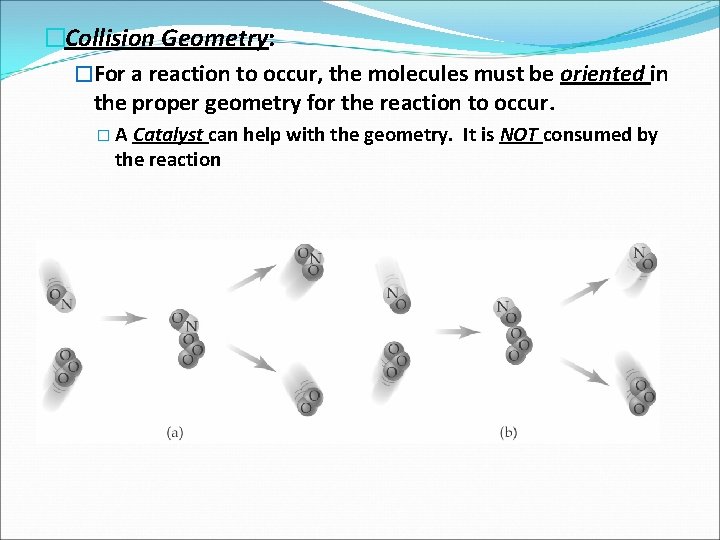
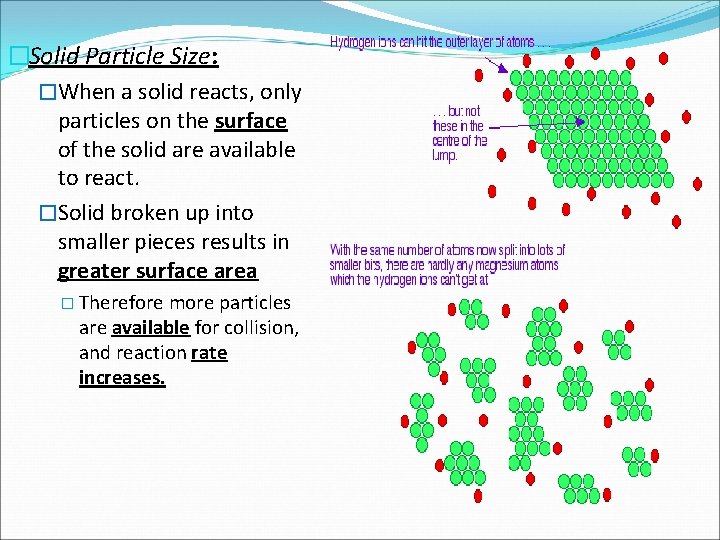
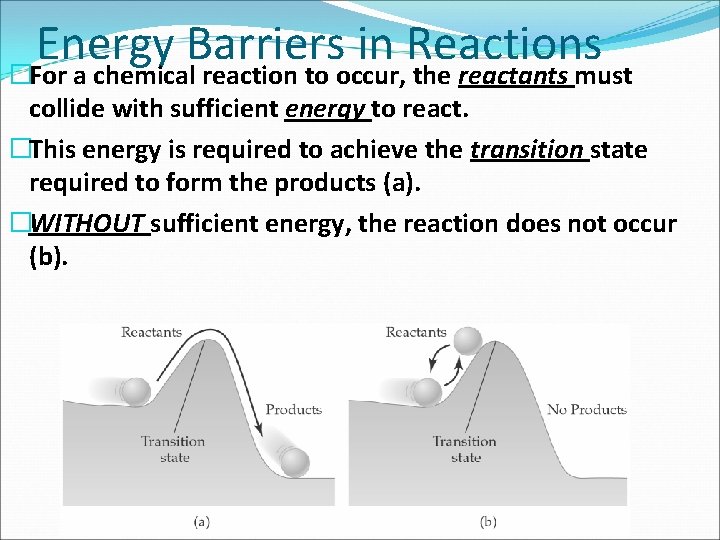
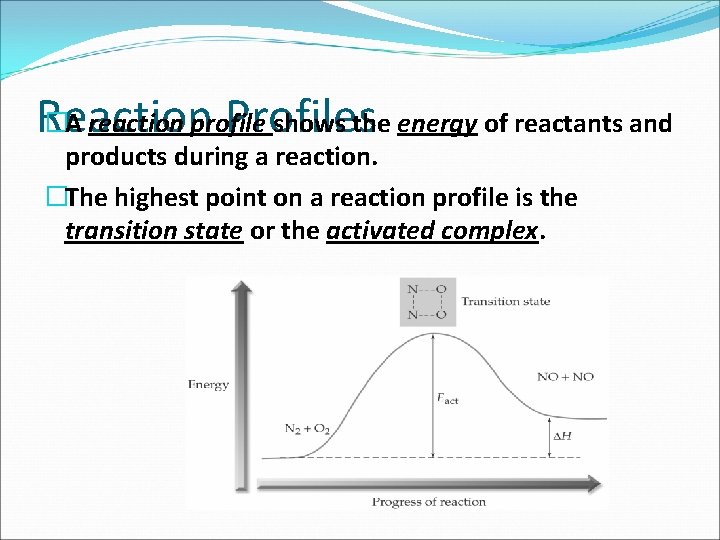
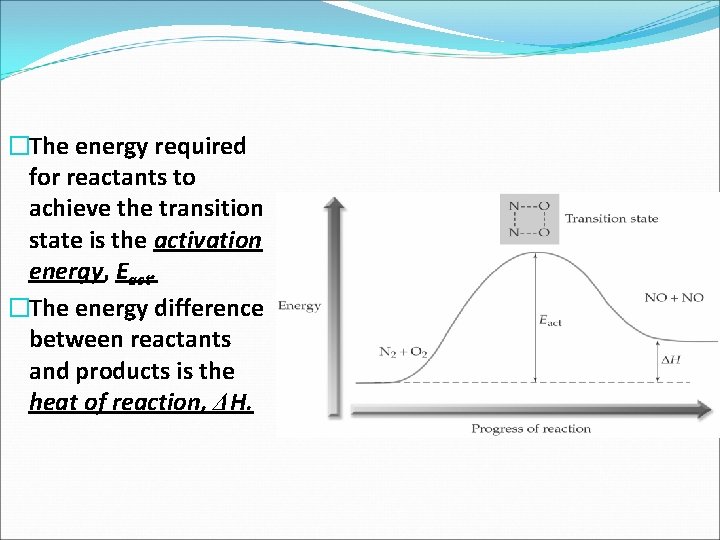
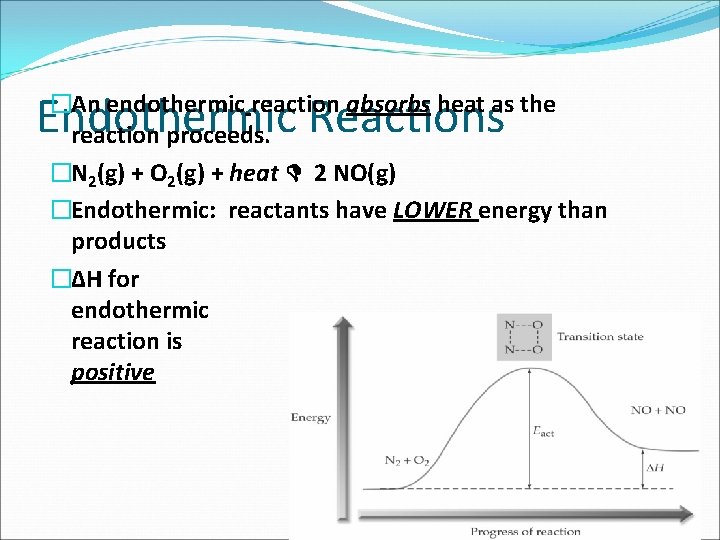
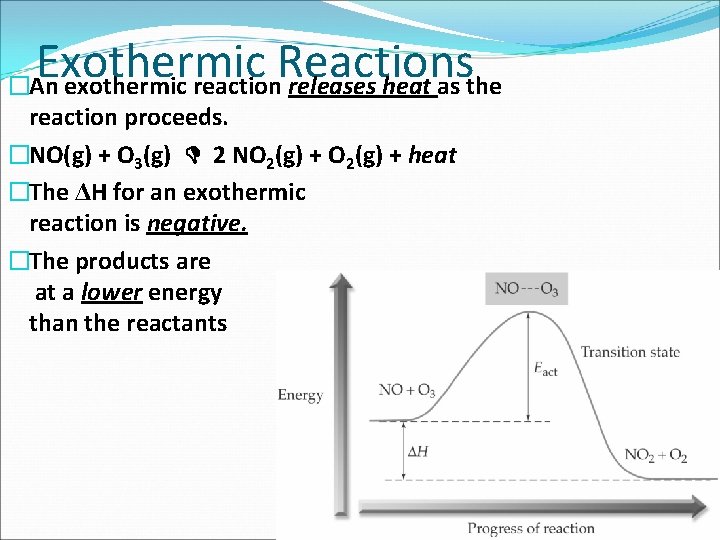
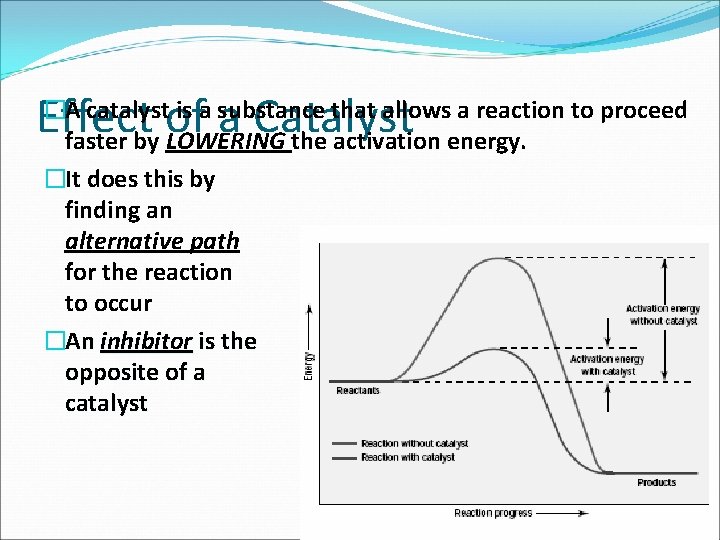
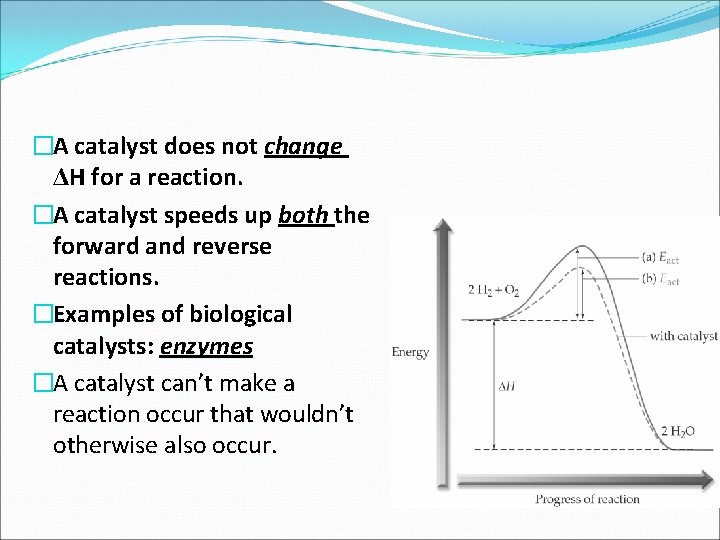
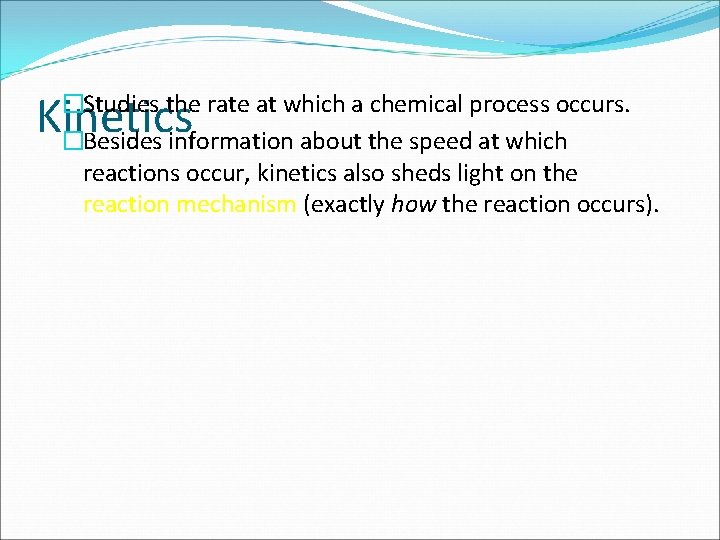
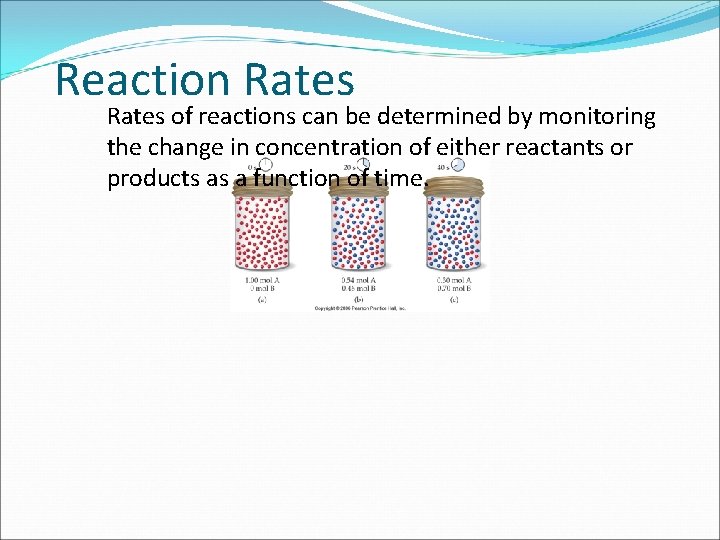
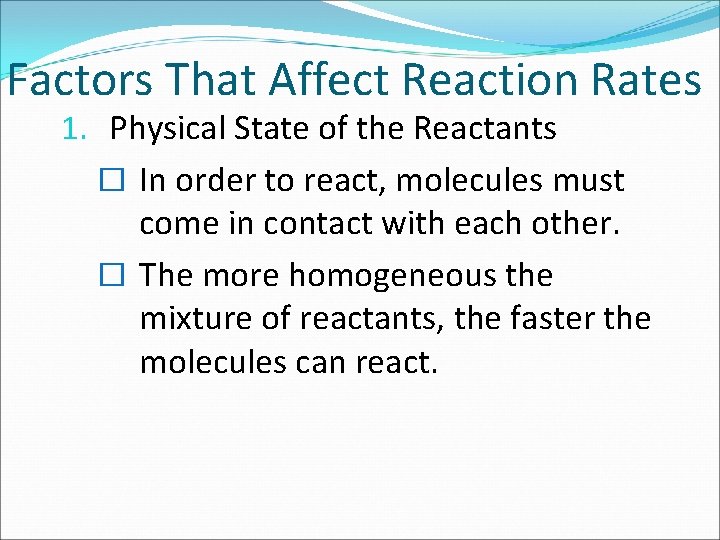
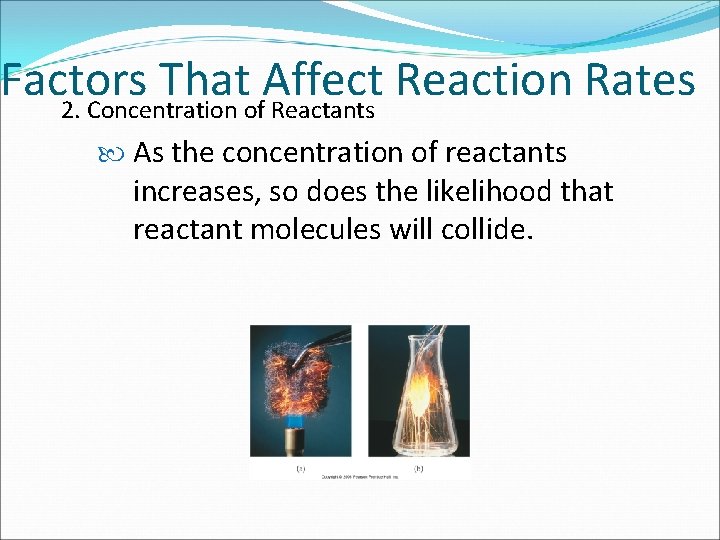
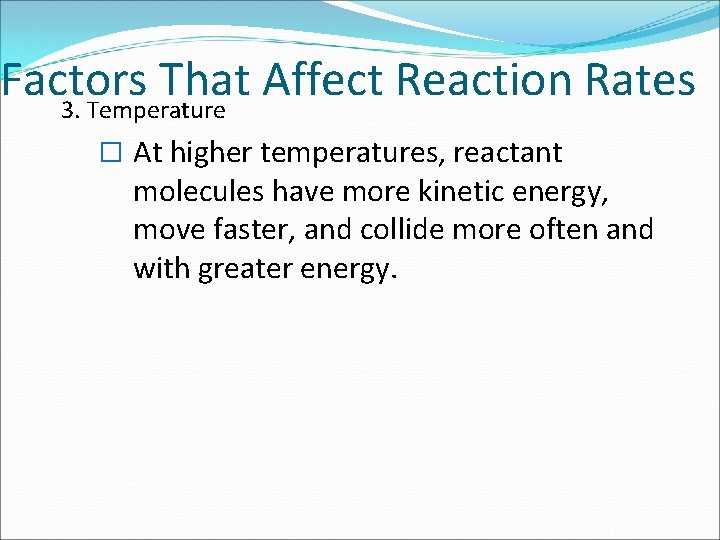
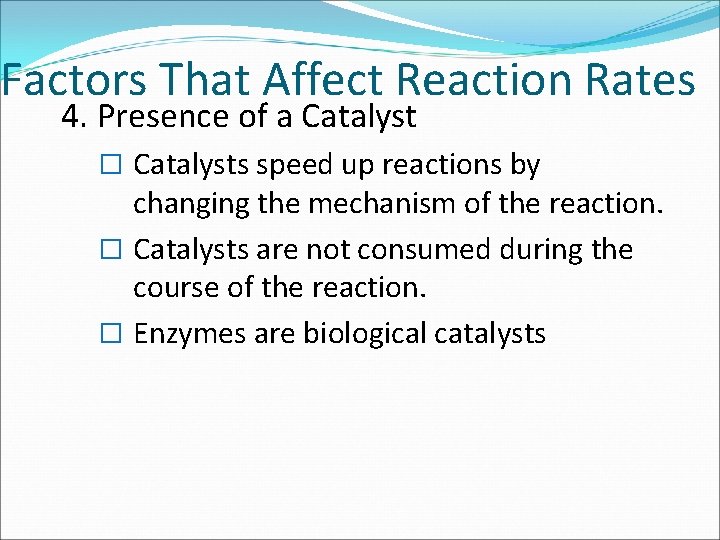
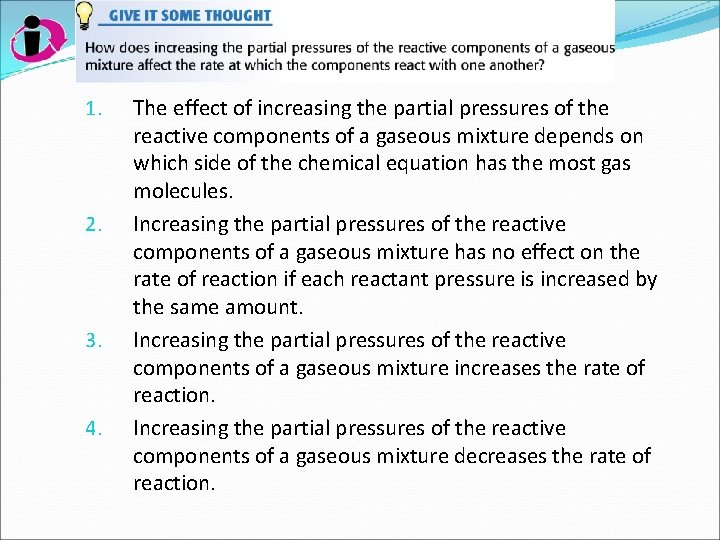
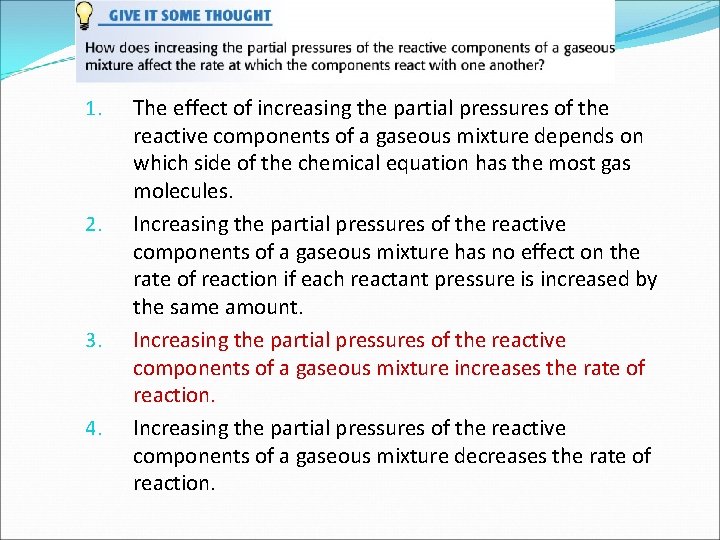
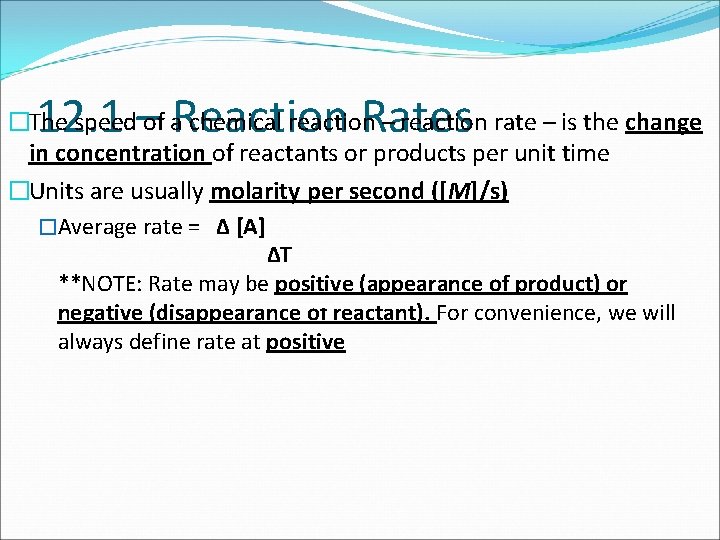
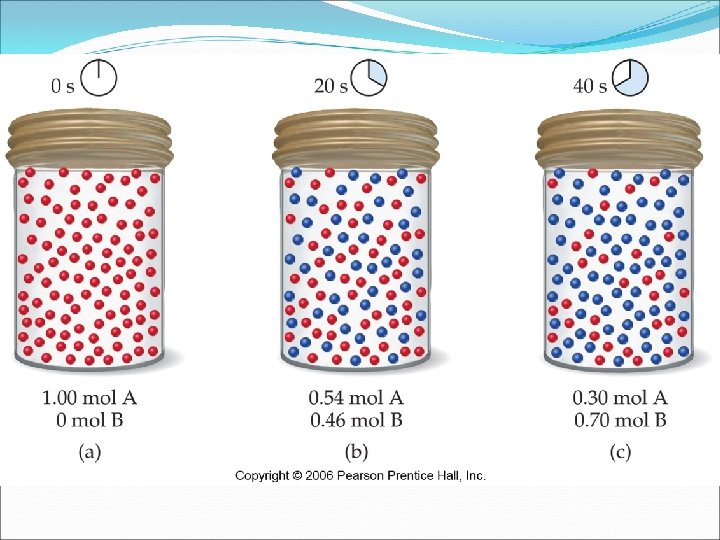
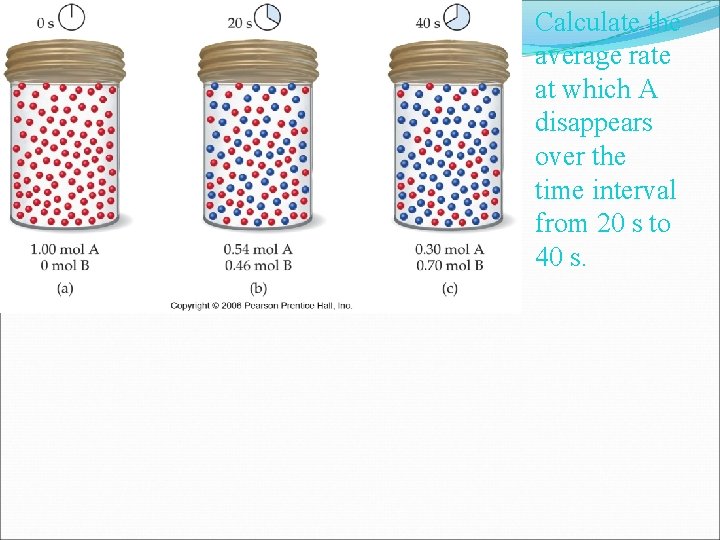
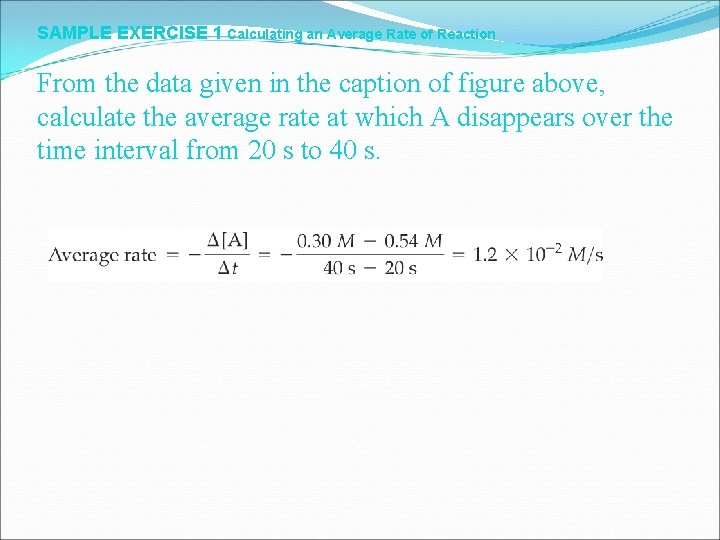
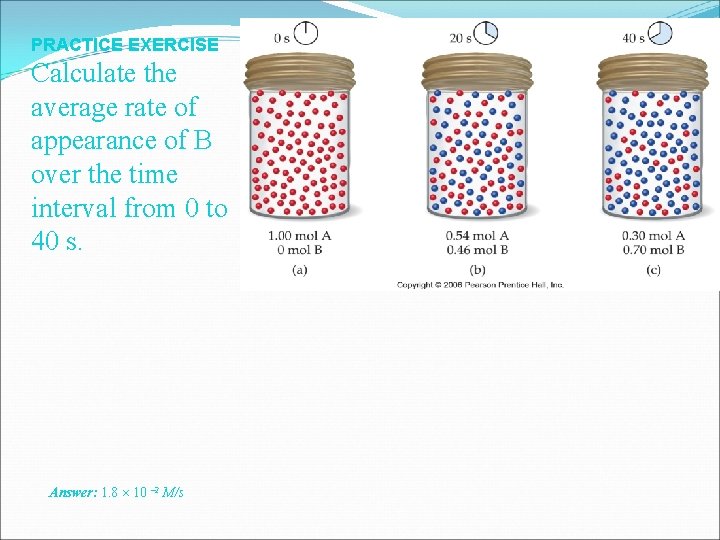
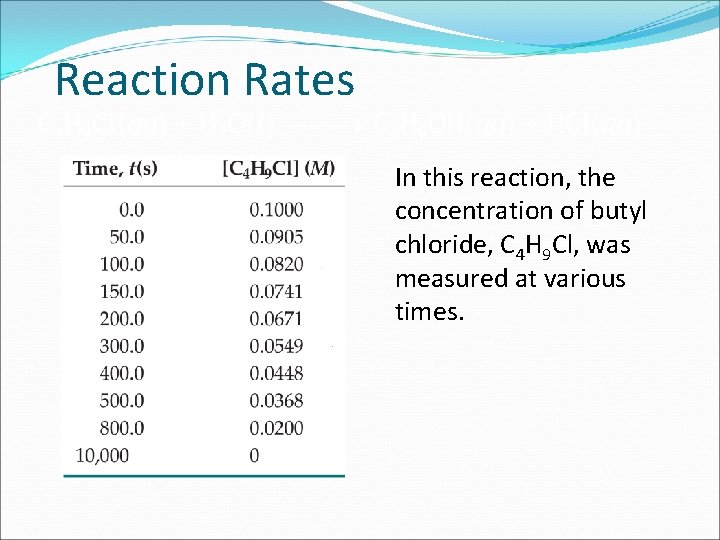
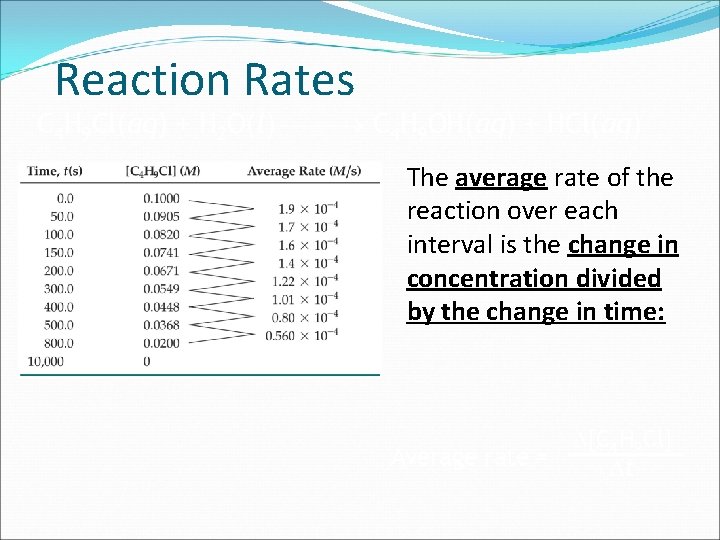
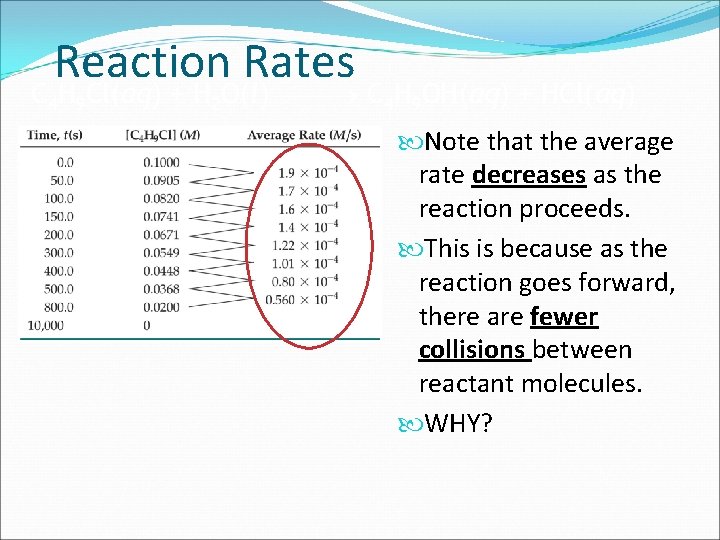
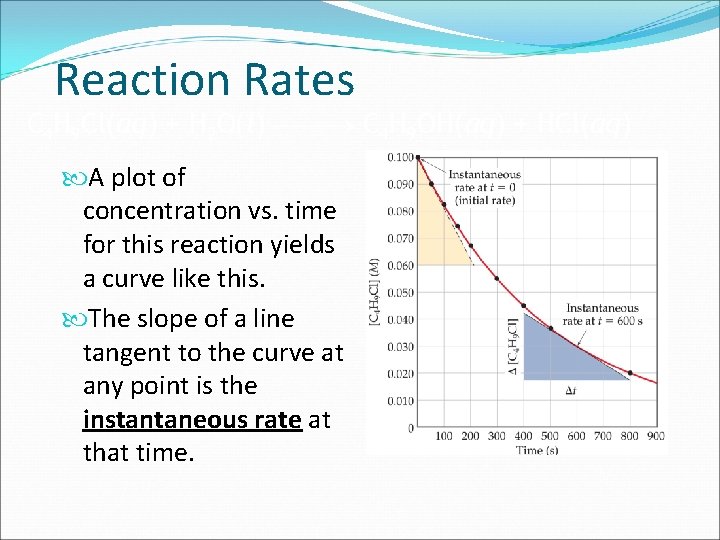
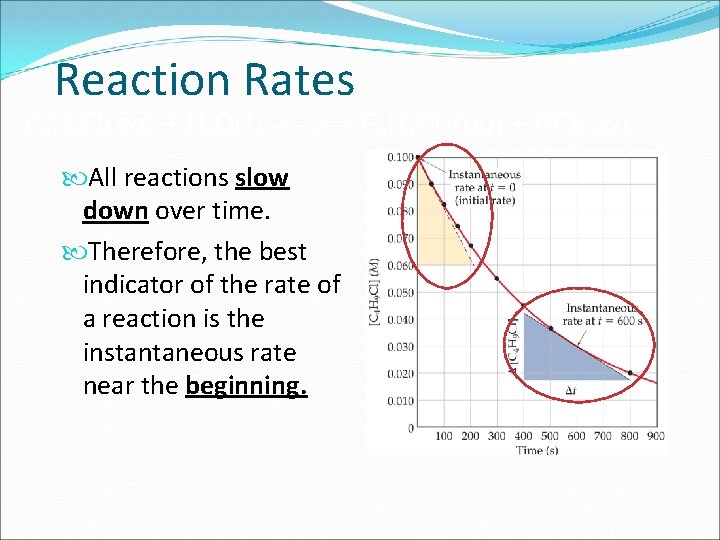
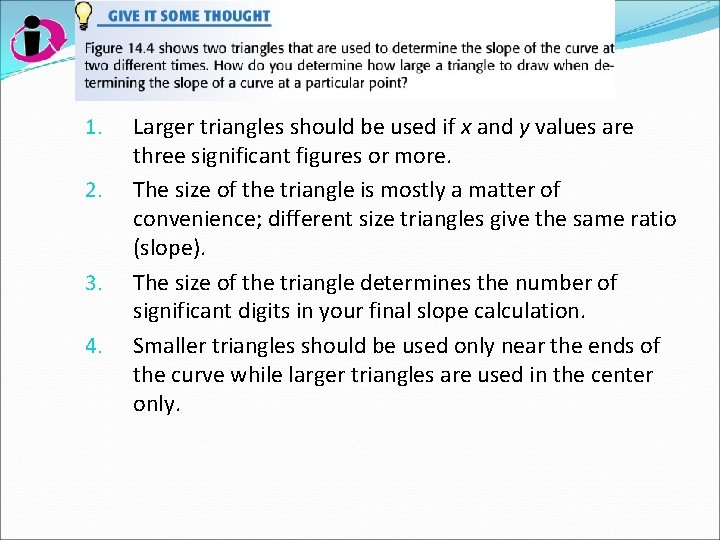
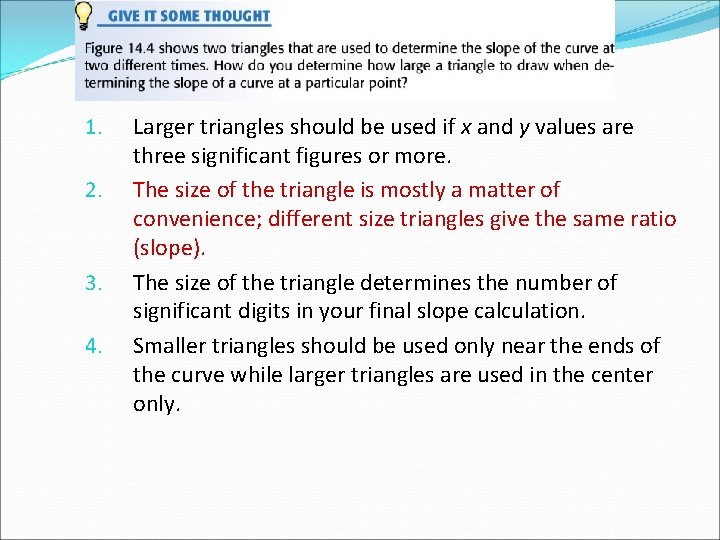
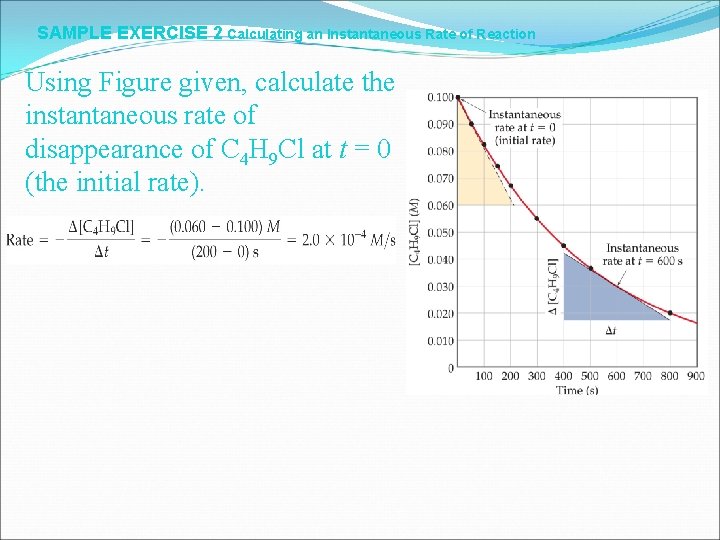
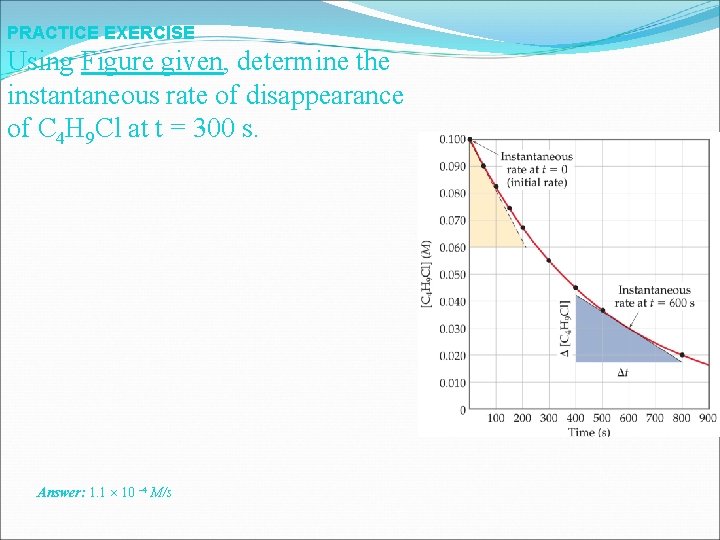
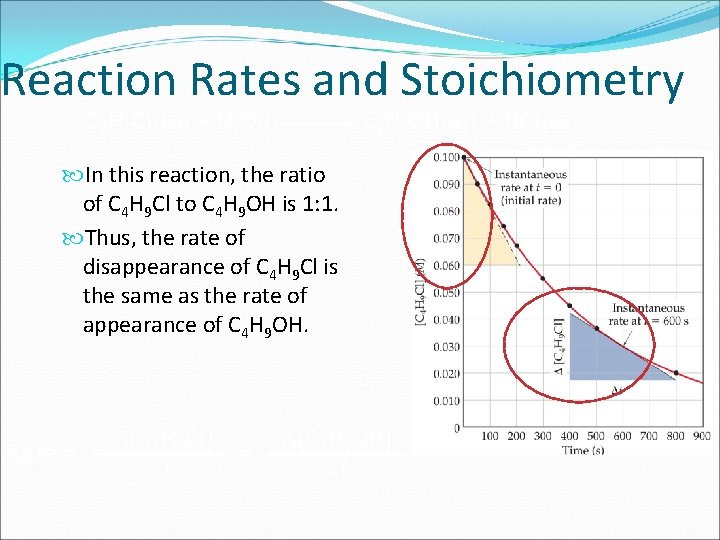
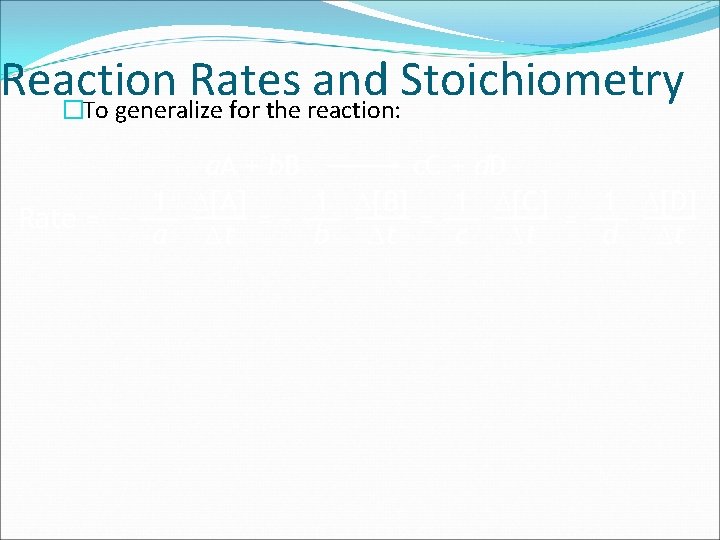
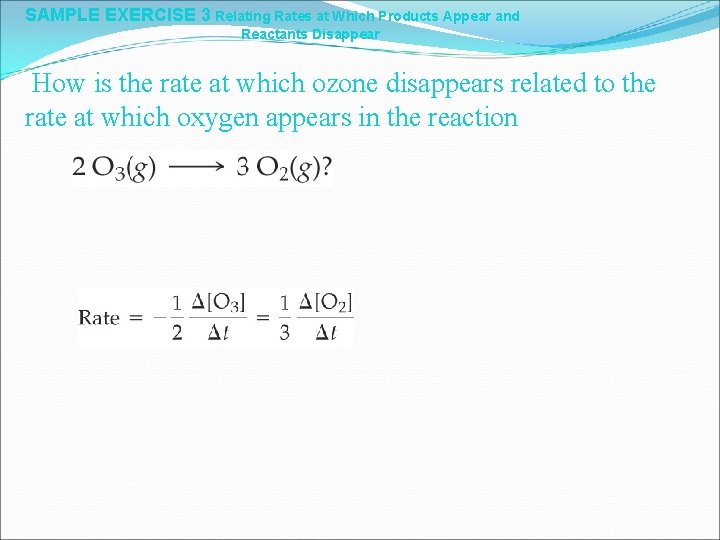
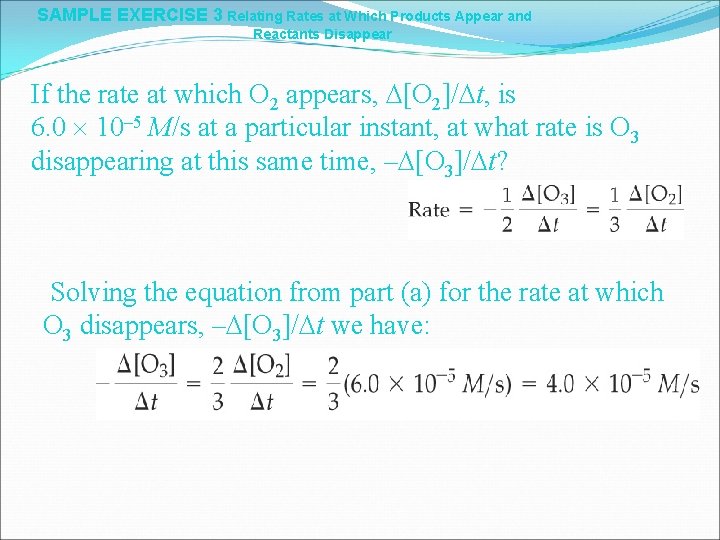
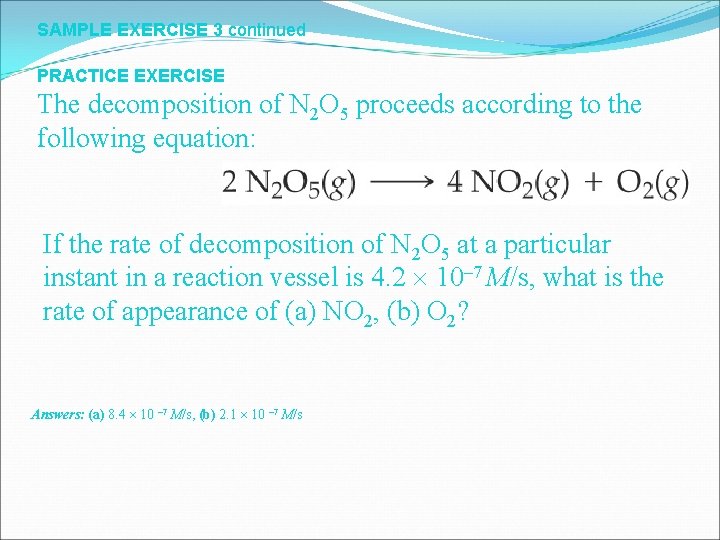
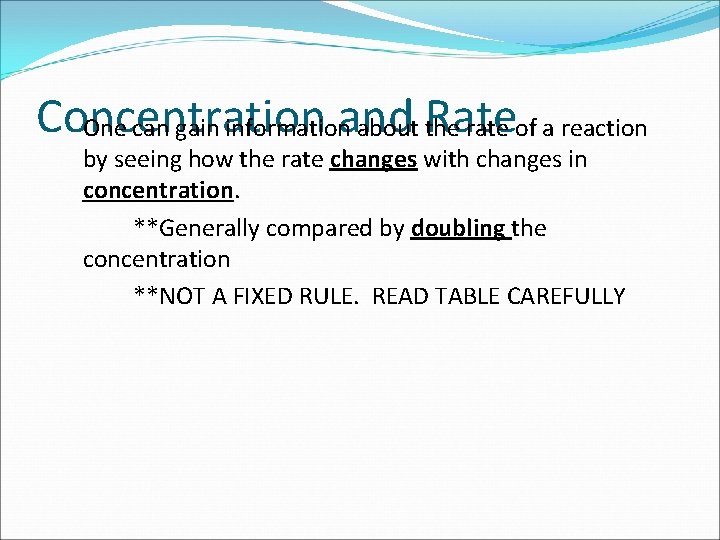
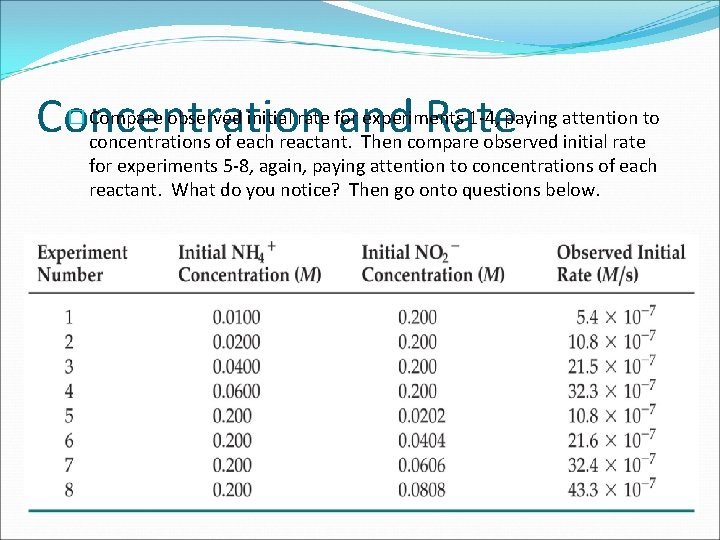
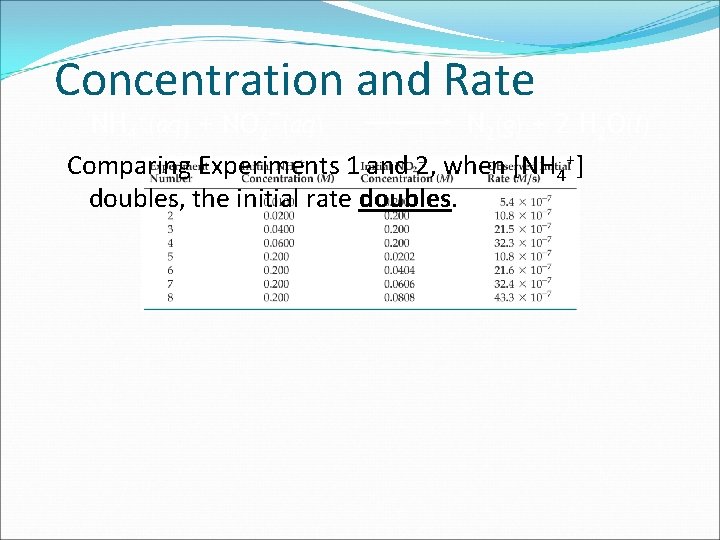
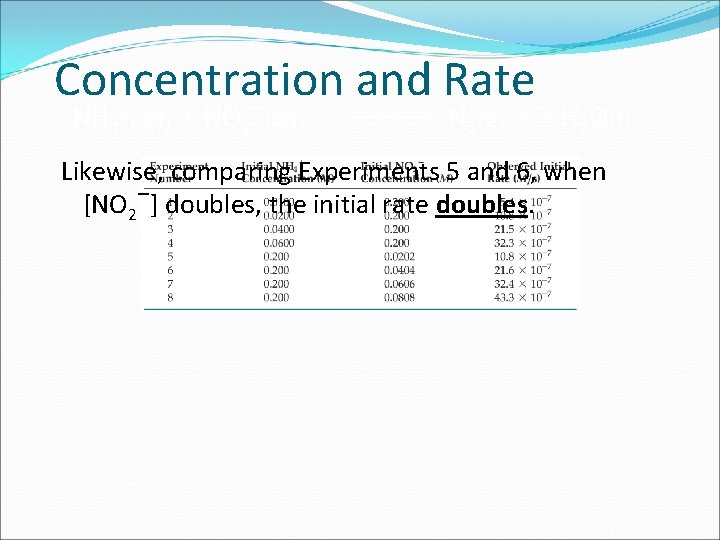
![This means Rate [NH 4+] Translation: rate is DIRECTLY proportionate to concentration of This means Rate [NH 4+] Translation: rate is DIRECTLY proportionate to concentration of](https://slidetodoc.com/presentation_image_h/1c9abc1fe8d10b8ee4aadf33e9ca8558/image-62.jpg)
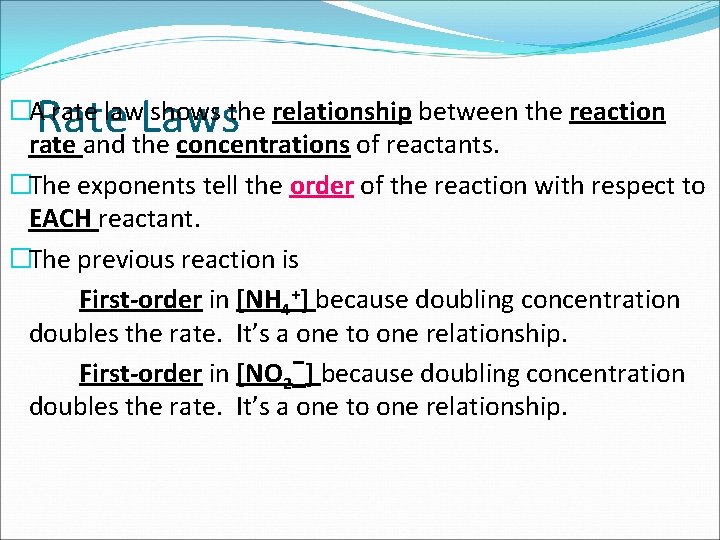
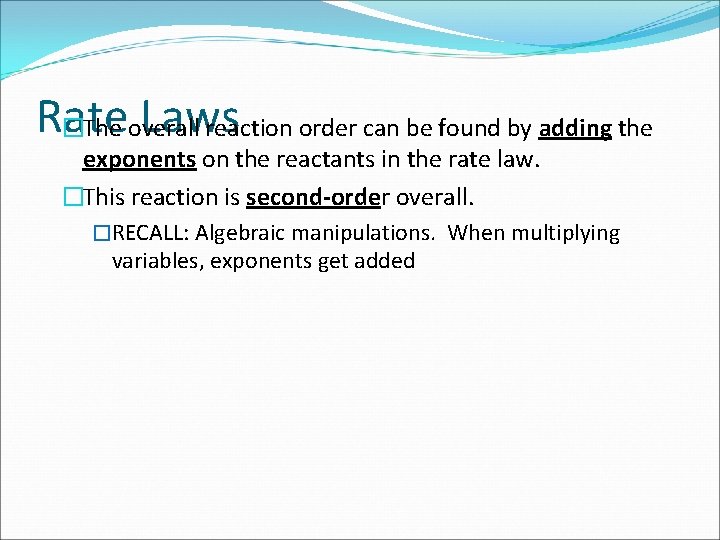
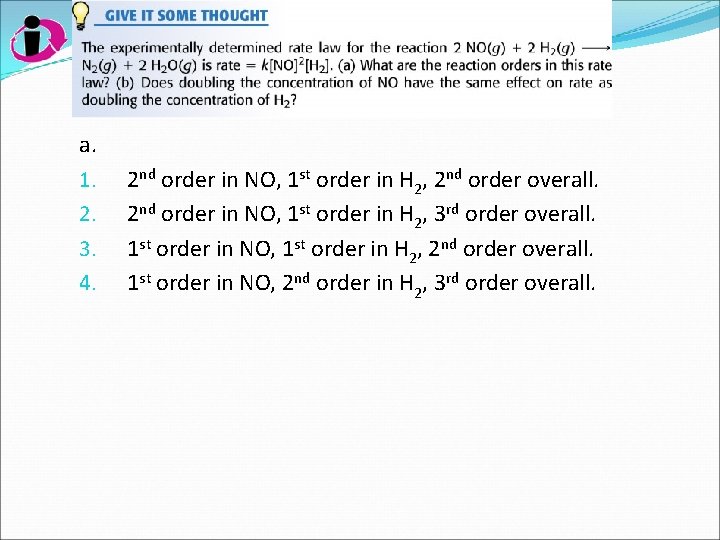
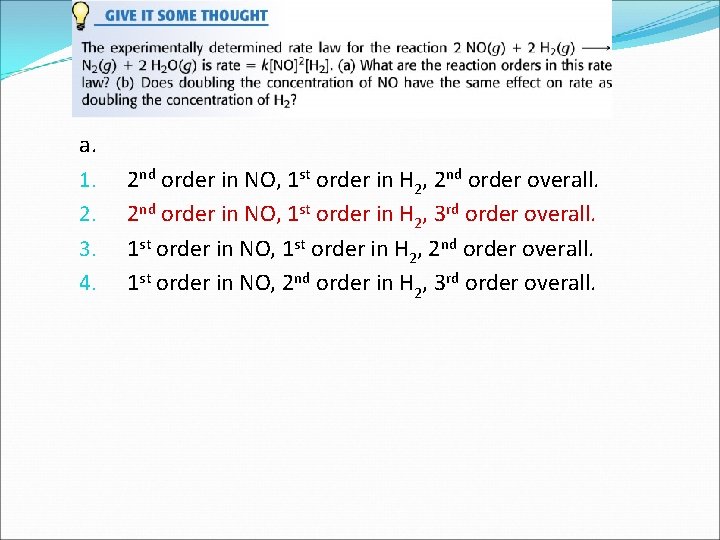
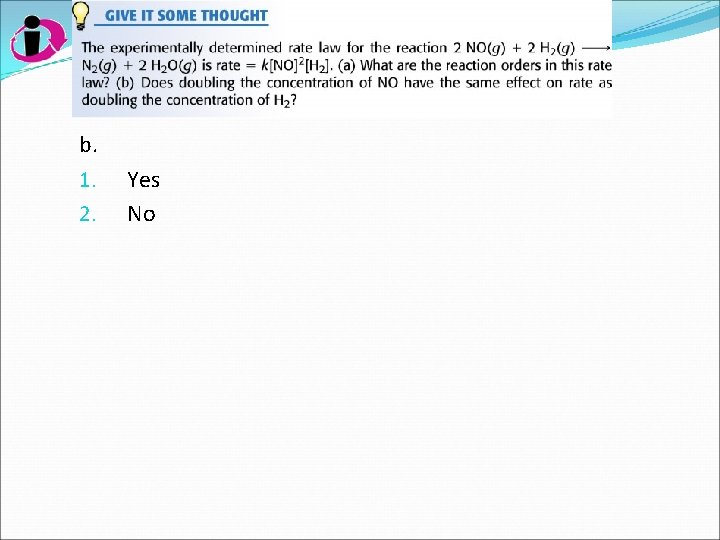
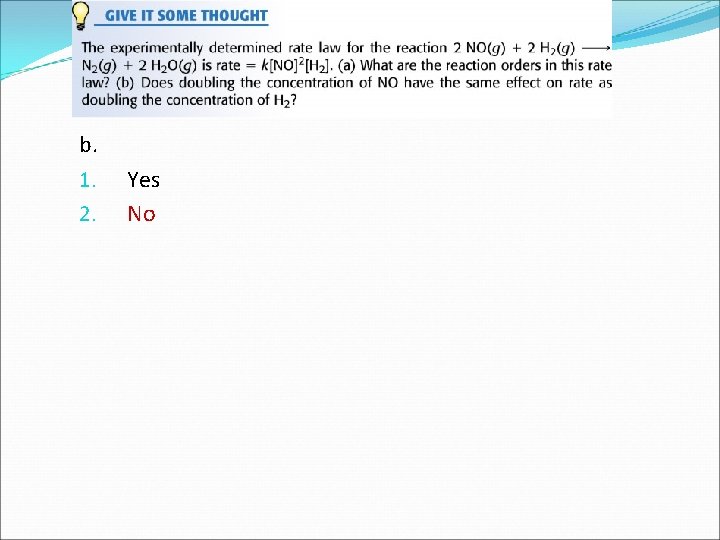
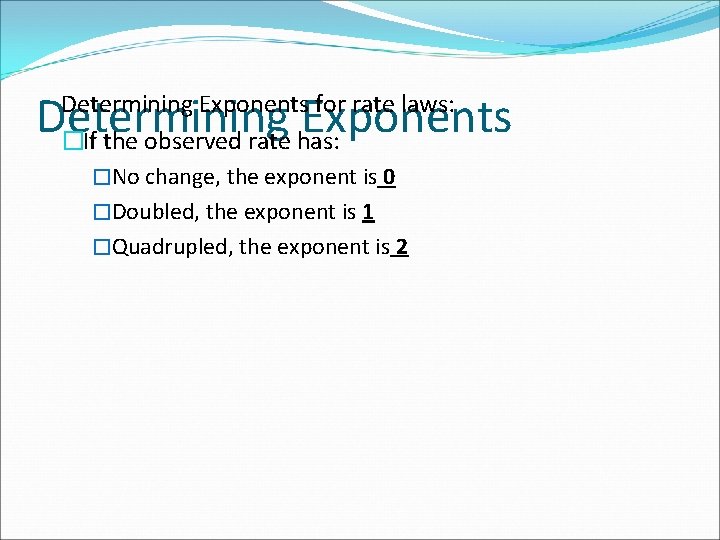
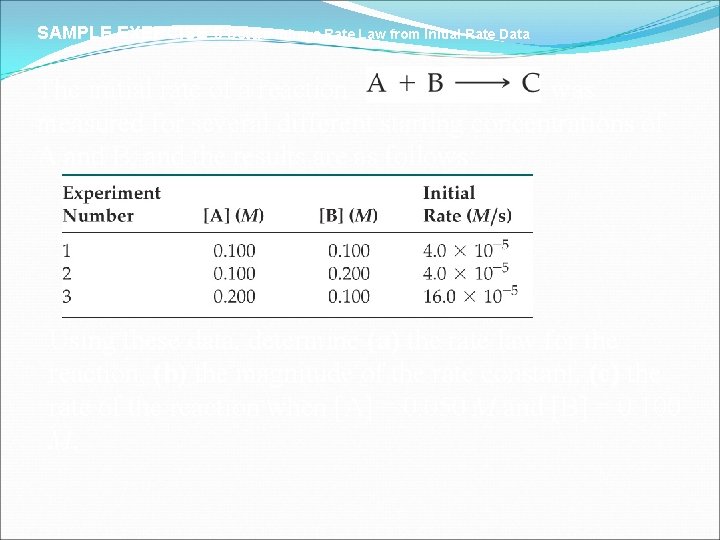
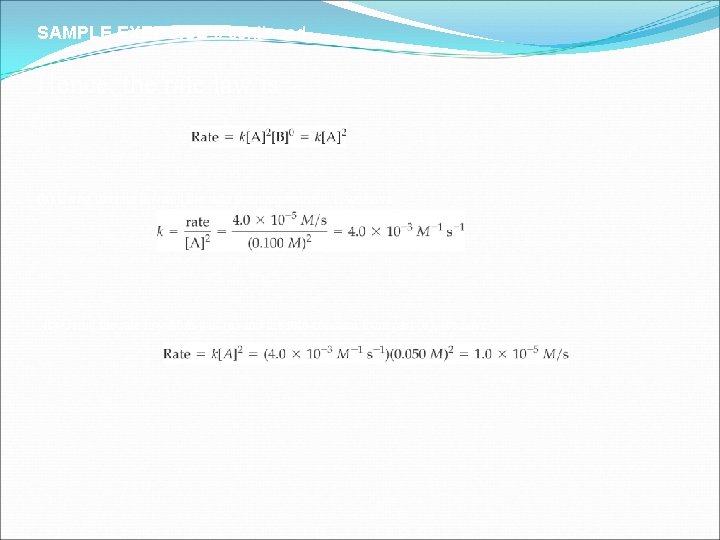
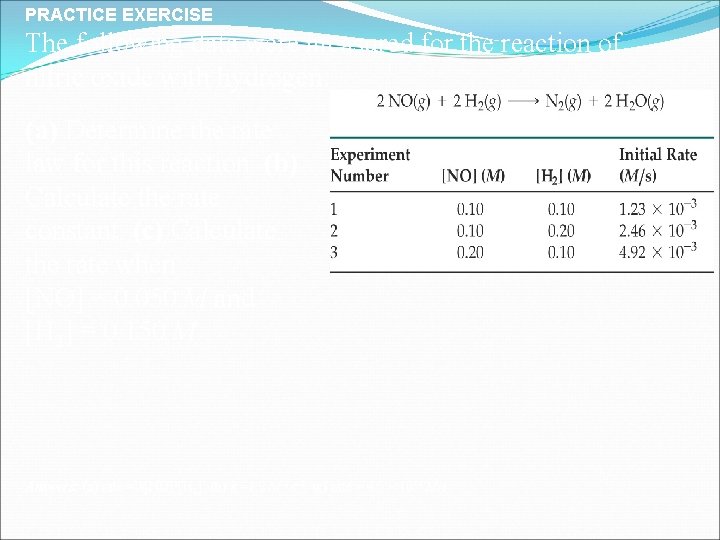
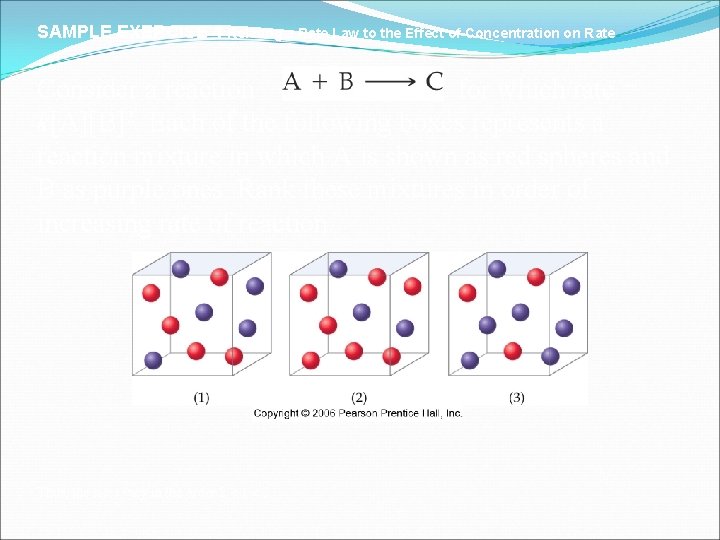
![PRACTICE EXERCISE Assuming that rate = k[A][B], rank the mixtures represented in this Sample PRACTICE EXERCISE Assuming that rate = k[A][B], rank the mixtures represented in this Sample](https://slidetodoc.com/presentation_image_h/1c9abc1fe8d10b8ee4aadf33e9ca8558/image-74.jpg)
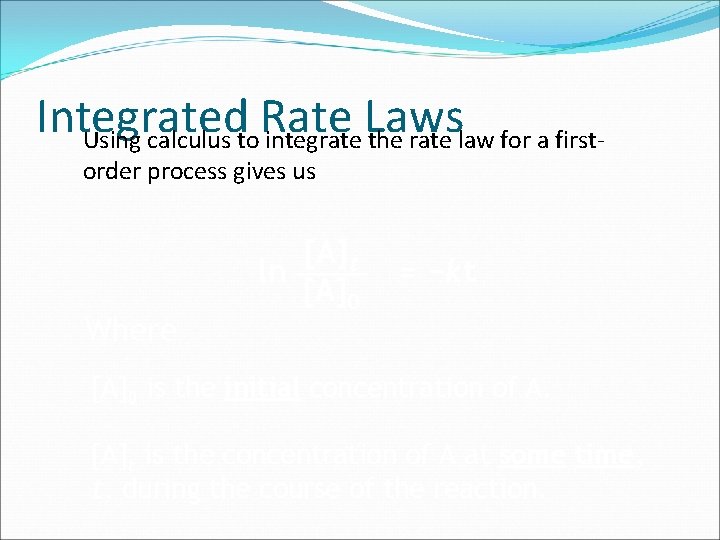
![Manipulating this equation Integrated Rateproduces… Laws [A]t ln = −kt [A]0 ln [A]t − Manipulating this equation Integrated Rateproduces… Laws [A]t ln = −kt [A]0 ln [A]t −](https://slidetodoc.com/presentation_image_h/1c9abc1fe8d10b8ee4aadf33e9ca8558/image-76.jpg)
![First-Order Processes Therefore, if a reaction is first-order, a plot of ln [A] vs. First-Order Processes Therefore, if a reaction is first-order, a plot of ln [A] vs.](https://slidetodoc.com/presentation_image_h/1c9abc1fe8d10b8ee4aadf33e9ca8558/image-77.jpg)
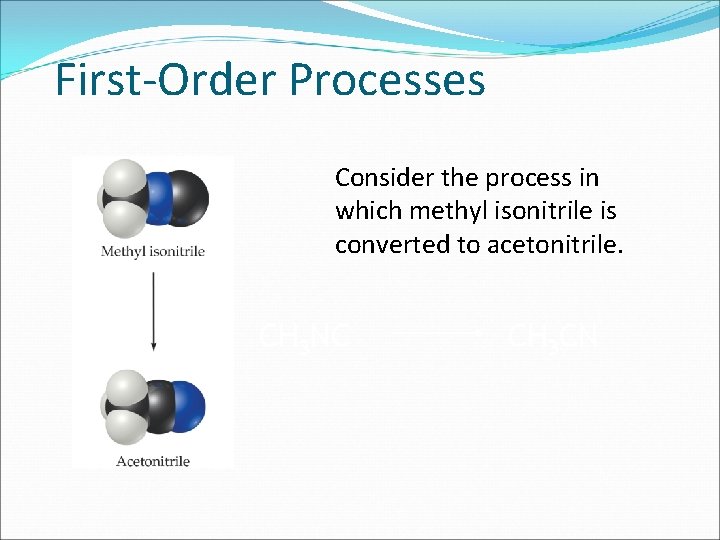
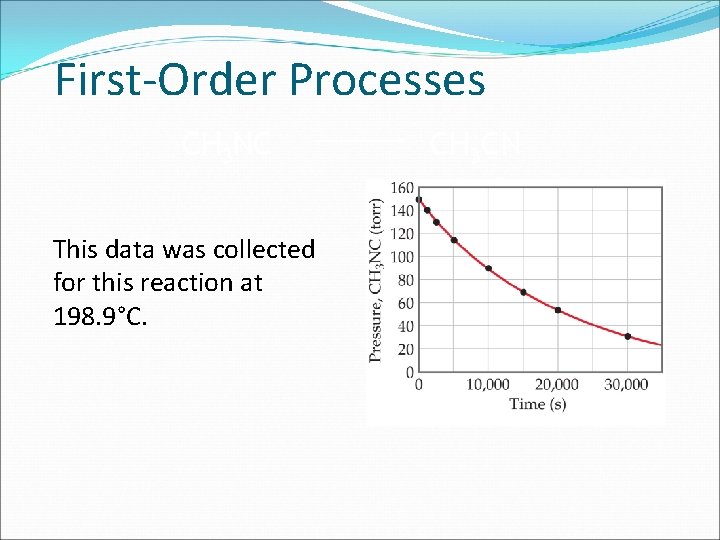
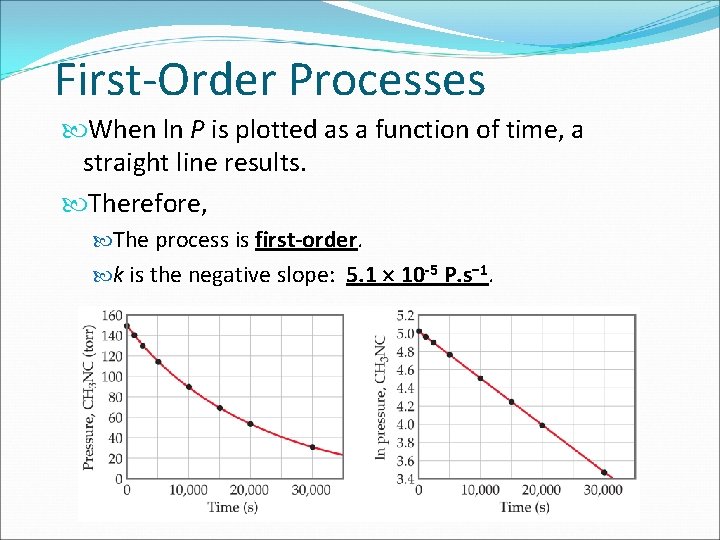
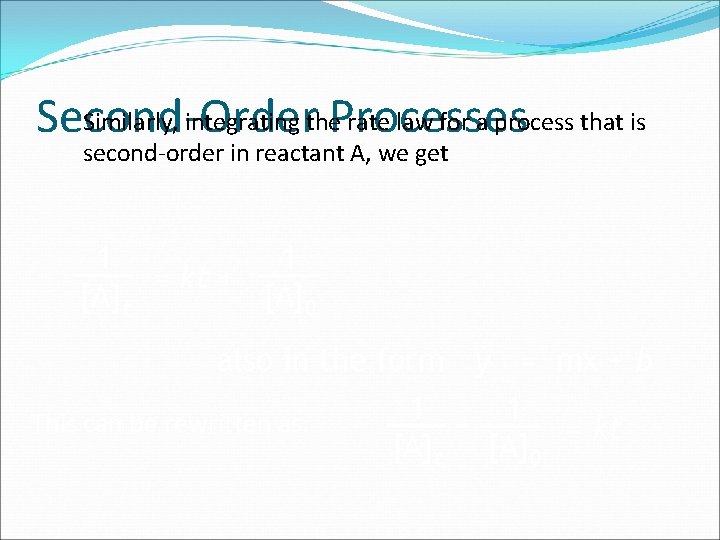
![Second-Order Processes 1 1 [A]t = kt + [A]0 So if a process is Second-Order Processes 1 1 [A]t = kt + [A]0 So if a process is](https://slidetodoc.com/presentation_image_h/1c9abc1fe8d10b8ee4aadf33e9ca8558/image-82.jpg)
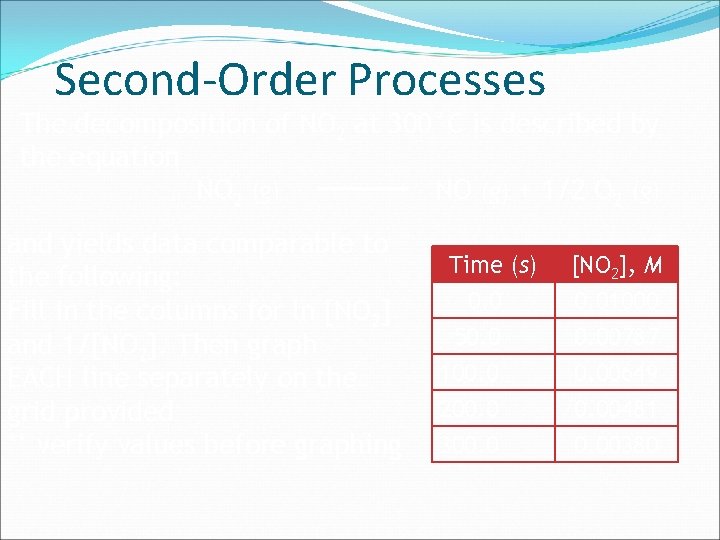
![Time (s) [NO 2], M ln [NO 2] 1/[NO 2] 0. 01000 − 4. Time (s) [NO 2], M ln [NO 2] 1/[NO 2] 0. 01000 − 4.](https://slidetodoc.com/presentation_image_h/1c9abc1fe8d10b8ee4aadf33e9ca8558/image-84.jpg)
![Second-Order Processes • Graphing ln [NO 2] vs. t yields: • The plot is Second-Order Processes • Graphing ln [NO 2] vs. t yields: • The plot is](https://slidetodoc.com/presentation_image_h/1c9abc1fe8d10b8ee4aadf33e9ca8558/image-85.jpg)
![Second-Order Processes • Graphing ln 1/[NO 2] vs. t, however, gives this plot. Time Second-Order Processes • Graphing ln 1/[NO 2] vs. t, however, gives this plot. Time](https://slidetodoc.com/presentation_image_h/1c9abc1fe8d10b8ee4aadf33e9ca8558/image-86.jpg)
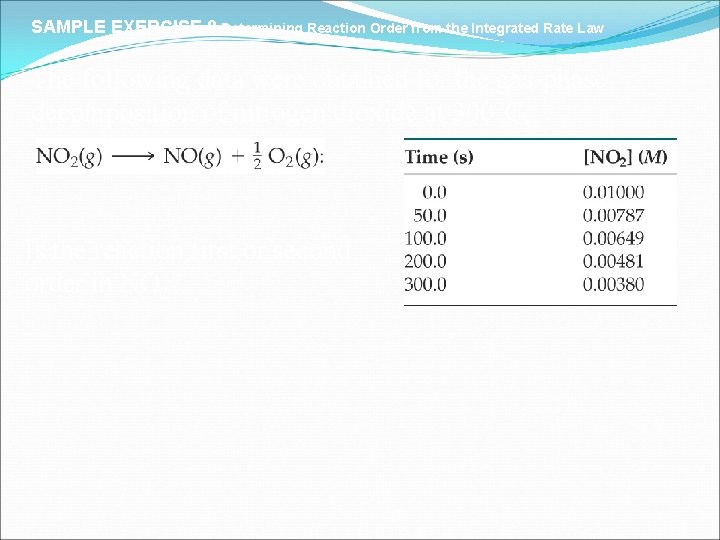
![SAMPLE EXERCISE 8 continued In order to graph ln[NO 2] and 1/[NO 2] against SAMPLE EXERCISE 8 continued In order to graph ln[NO 2] and 1/[NO 2] against](https://slidetodoc.com/presentation_image_h/1c9abc1fe8d10b8ee4aadf33e9ca8558/image-88.jpg)
![SAMPLE EXERCISE 8 continued As Figure below shows, only the plot of 1/[NO 2] SAMPLE EXERCISE 8 continued As Figure below shows, only the plot of 1/[NO 2]](https://slidetodoc.com/presentation_image_h/1c9abc1fe8d10b8ee4aadf33e9ca8558/image-89.jpg)
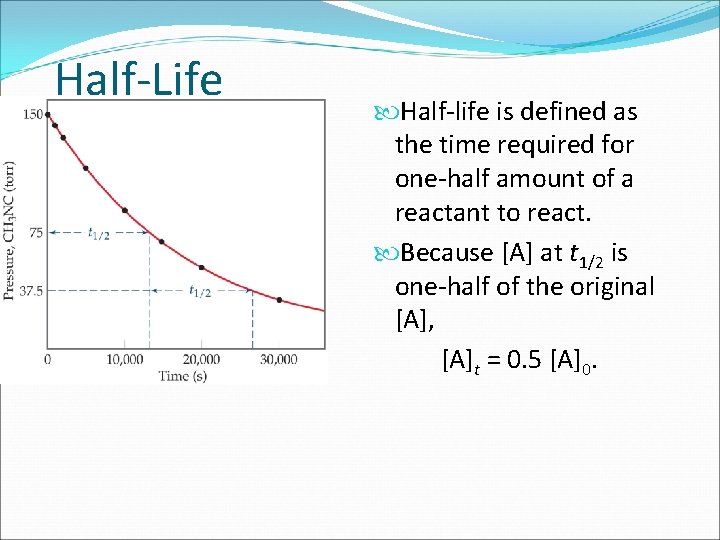
![For a first-order process, this becomes Half-Life 0. 5 [A]0 ln = −kt 1/2 For a first-order process, this becomes Half-Life 0. 5 [A]0 ln = −kt 1/2](https://slidetodoc.com/presentation_image_h/1c9abc1fe8d10b8ee4aadf33e9ca8558/image-91.jpg)
![For a second-order process, Half-Life 1 1 = kt 1/2 + 0. 5 [A]0 For a second-order process, Half-Life 1 1 = kt 1/2 + 0. 5 [A]0](https://slidetodoc.com/presentation_image_h/1c9abc1fe8d10b8ee4aadf33e9ca8558/image-92.jpg)
![Graphs and Kinetic Data �Plotting concentration of reactant [REACTANT] (or other relevant value) against Graphs and Kinetic Data �Plotting concentration of reactant [REACTANT] (or other relevant value) against](https://slidetodoc.com/presentation_image_h/1c9abc1fe8d10b8ee4aadf33e9ca8558/image-93.jpg)
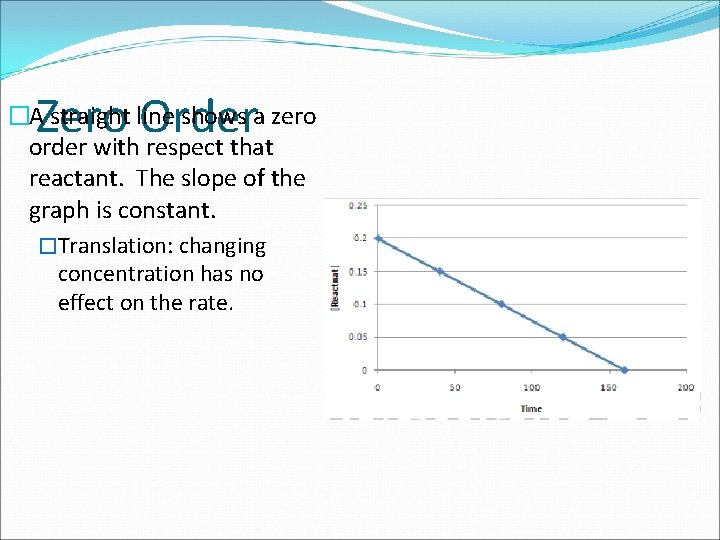
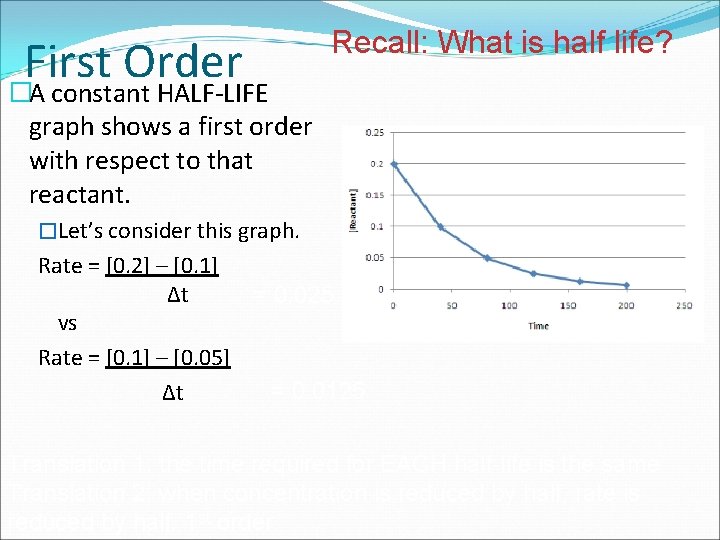
![Second order �Carefully note: curve of Rate = [0. 2] – [0. 1] ∆t Second order �Carefully note: curve of Rate = [0. 2] – [0. 1] ∆t](https://slidetodoc.com/presentation_image_h/1c9abc1fe8d10b8ee4aadf33e9ca8558/image-96.jpg)
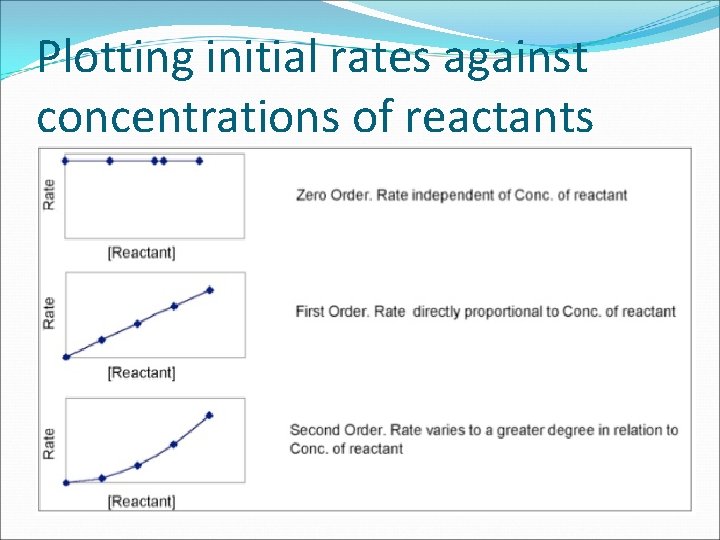
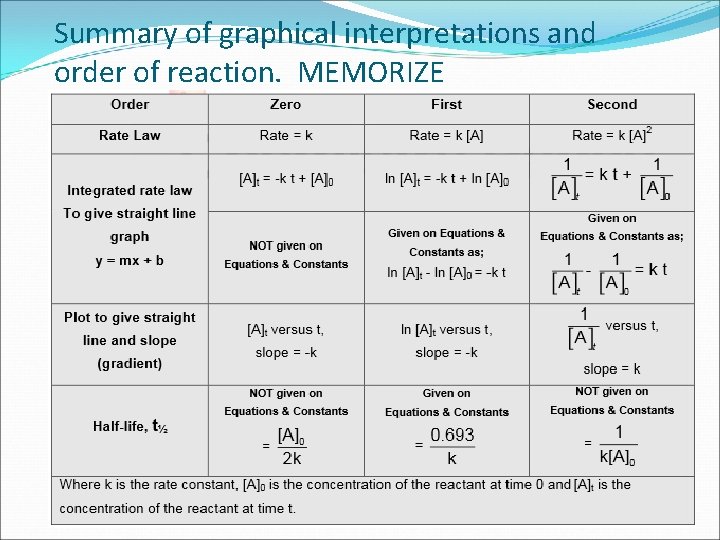
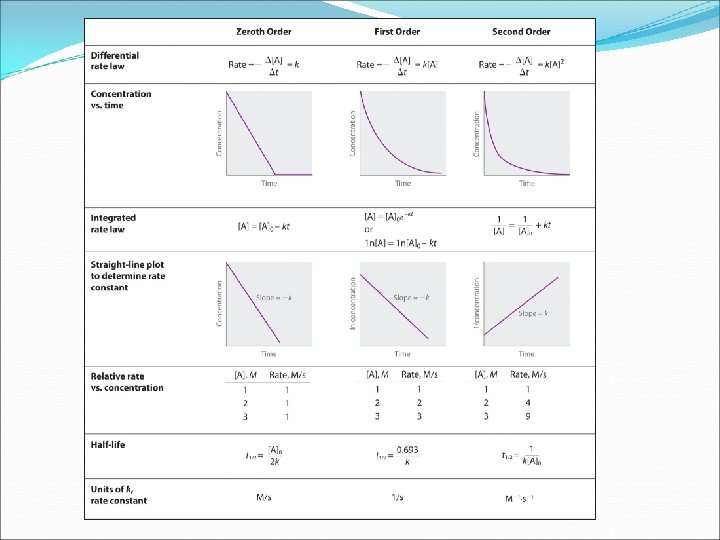
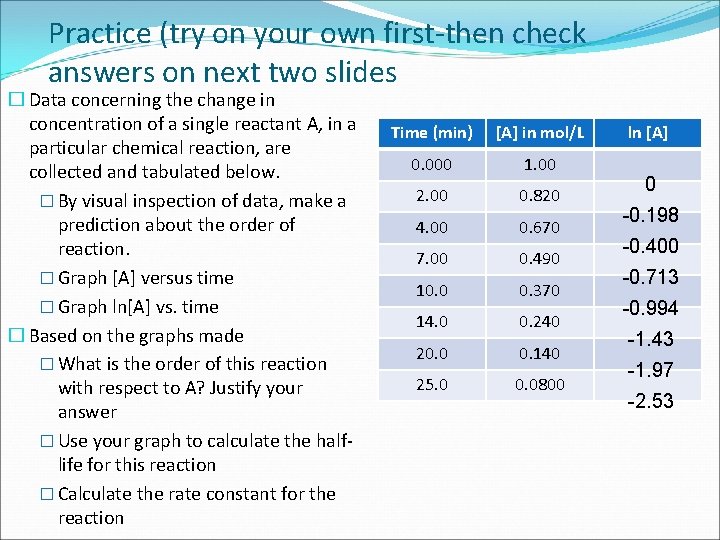
![1. 2 0 time vs. [A] 0 1 time ln[A] 20 10 vs. 15 1. 2 0 time vs. [A] 0 1 time ln[A] 20 10 vs. 15](https://slidetodoc.com/presentation_image_h/1c9abc1fe8d10b8ee4aadf33e9ca8558/image-101.jpg)
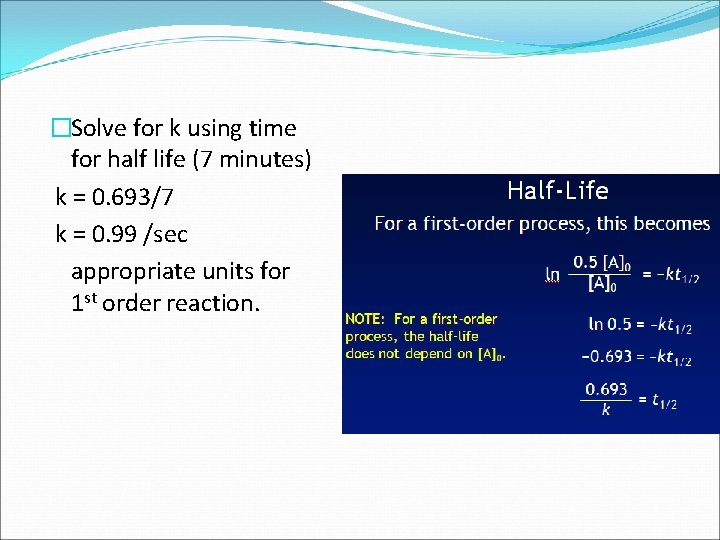
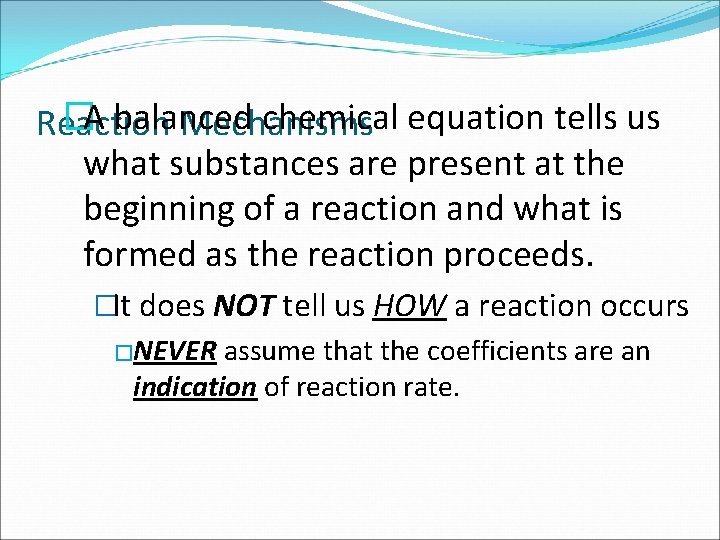
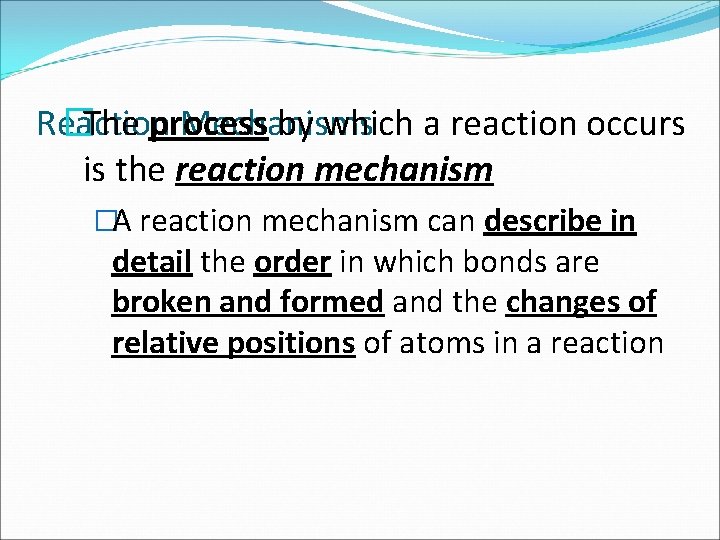
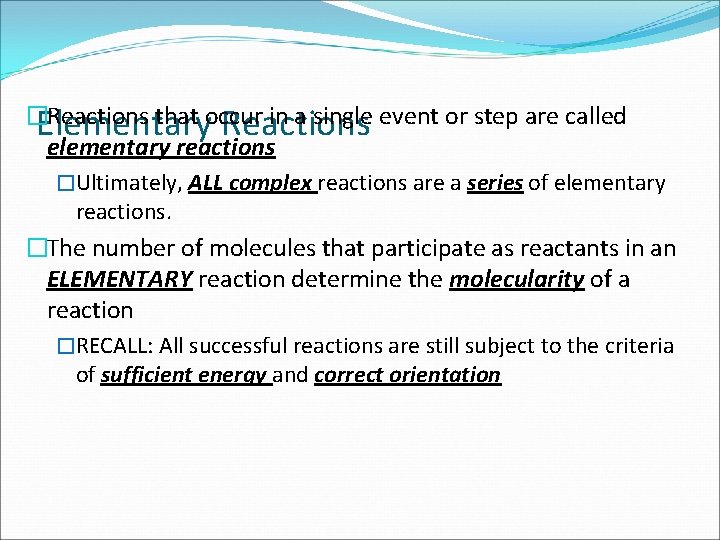
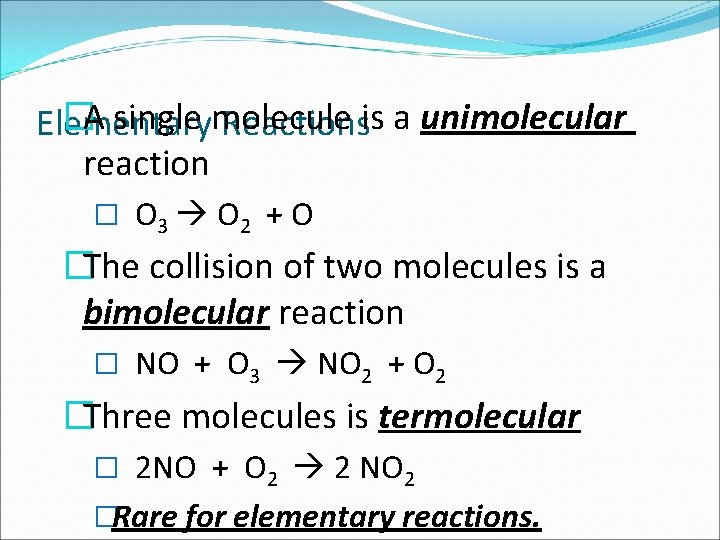
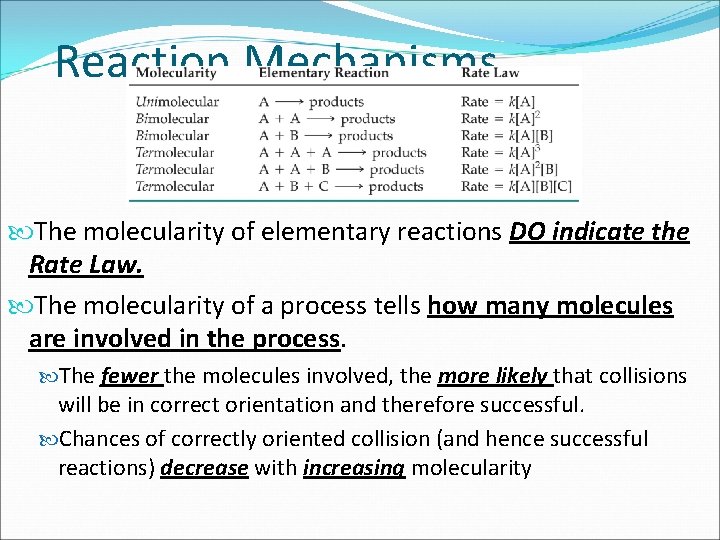
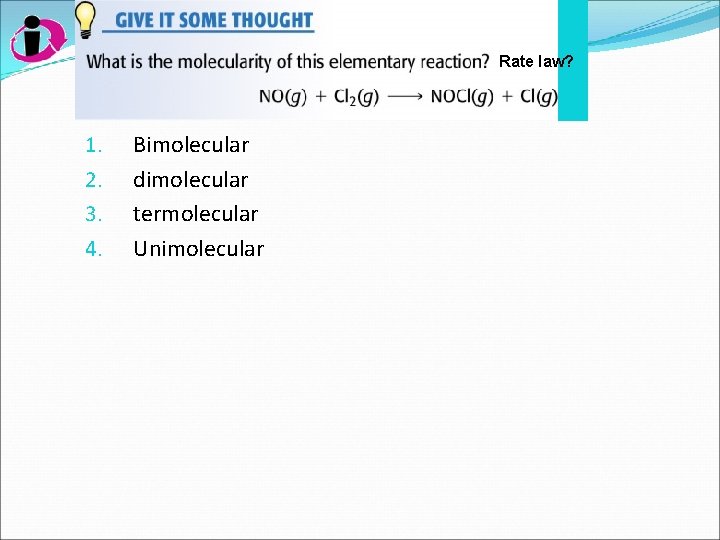
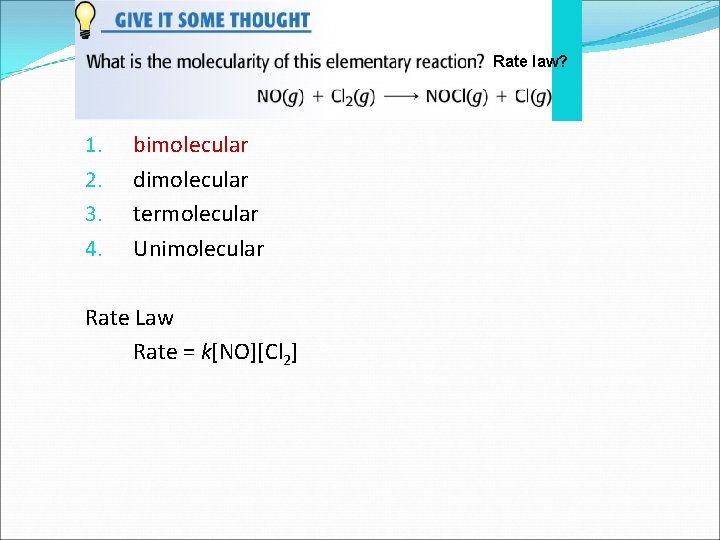
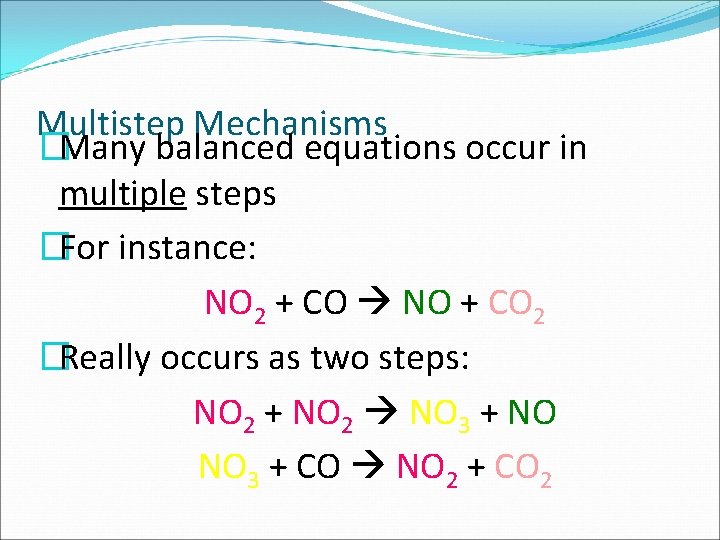
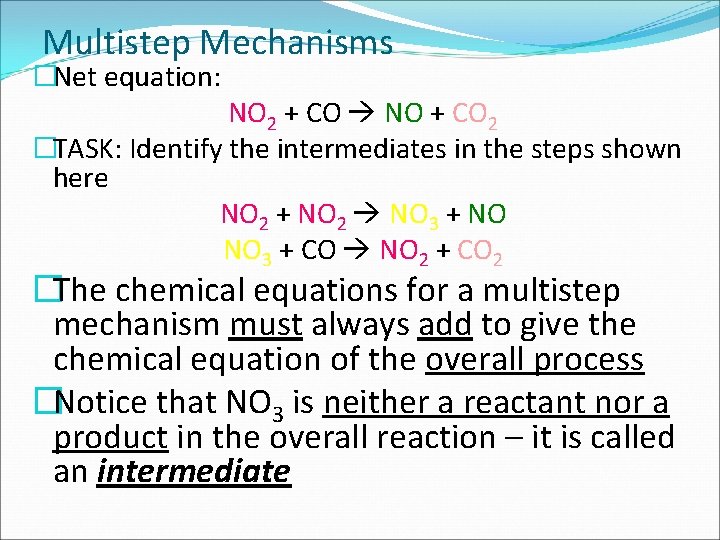
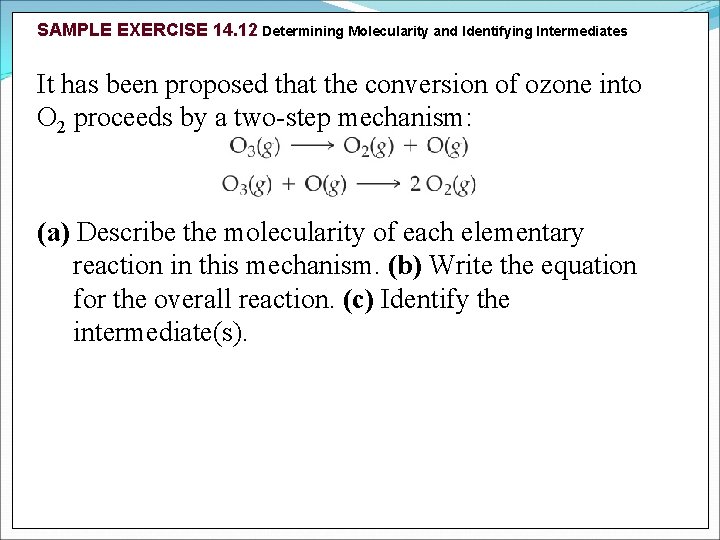
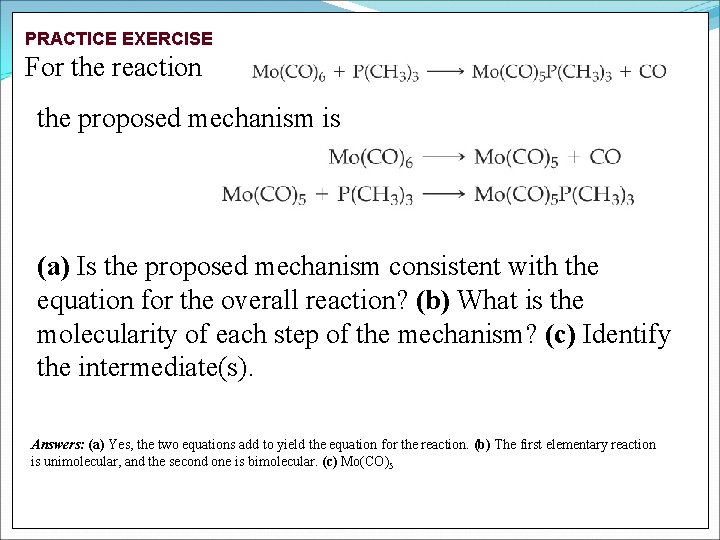
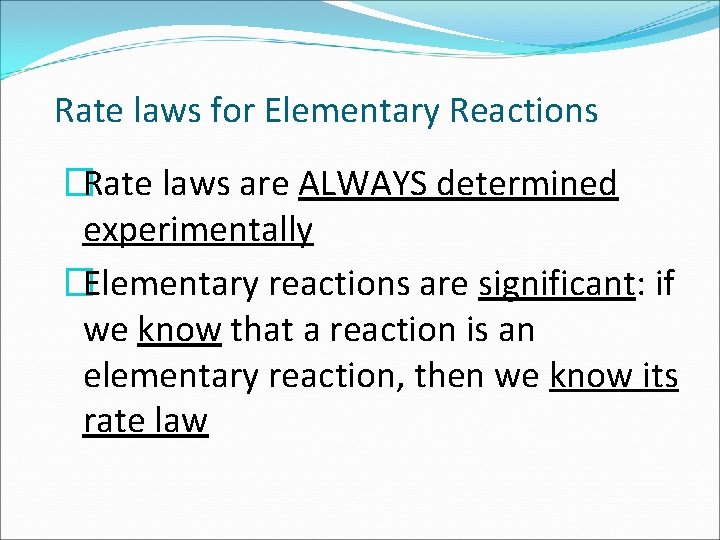
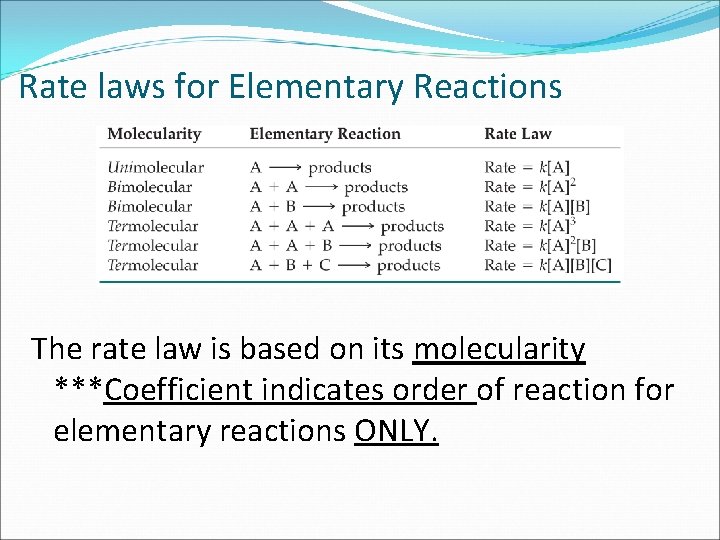
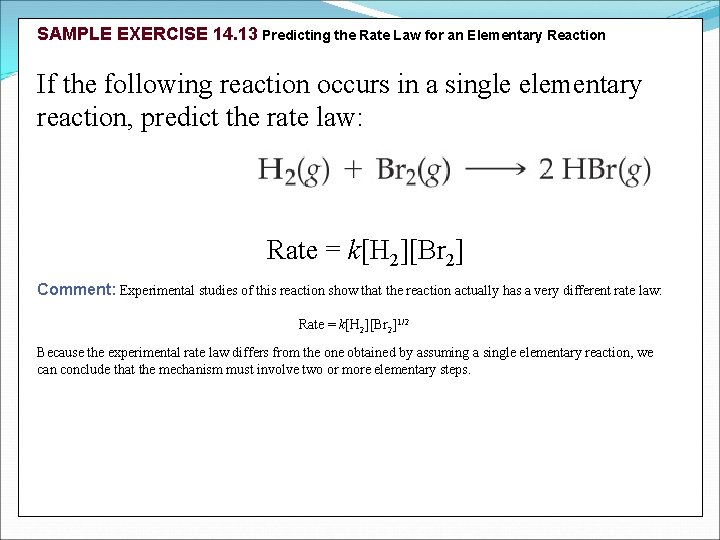
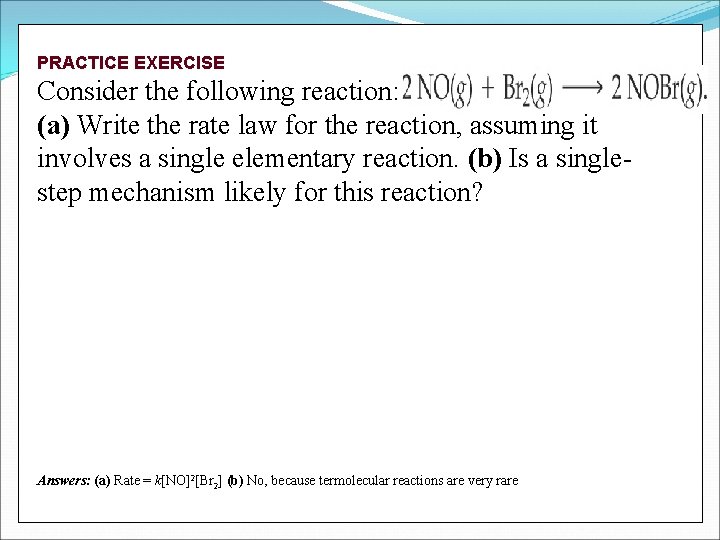
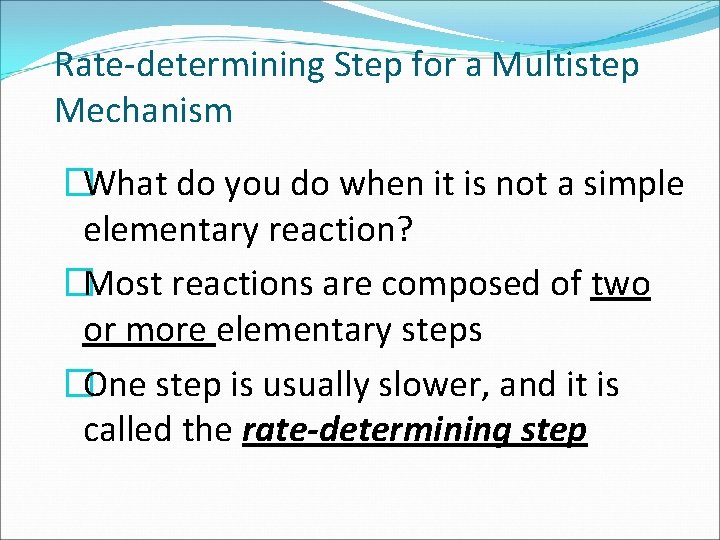
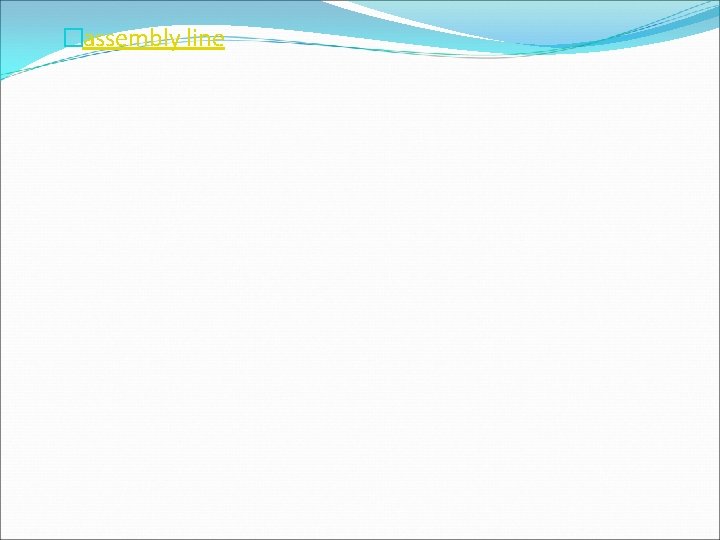
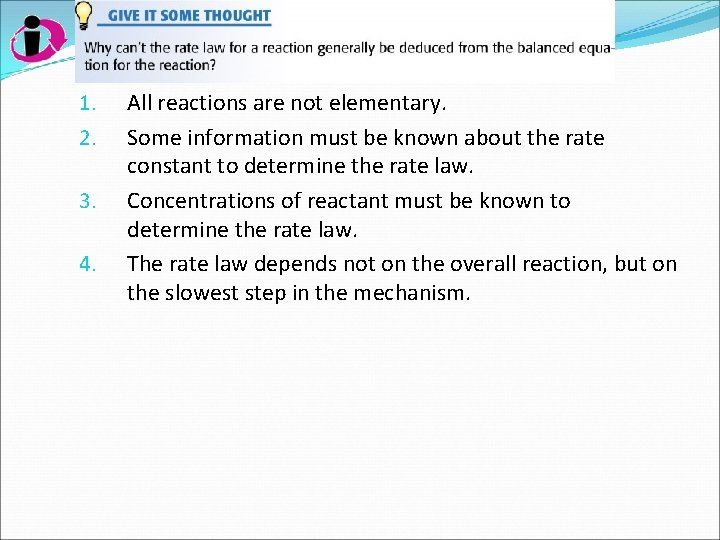
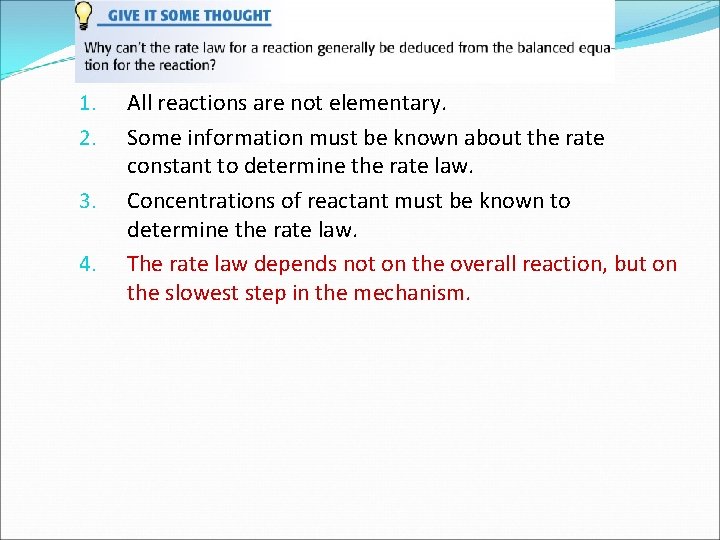
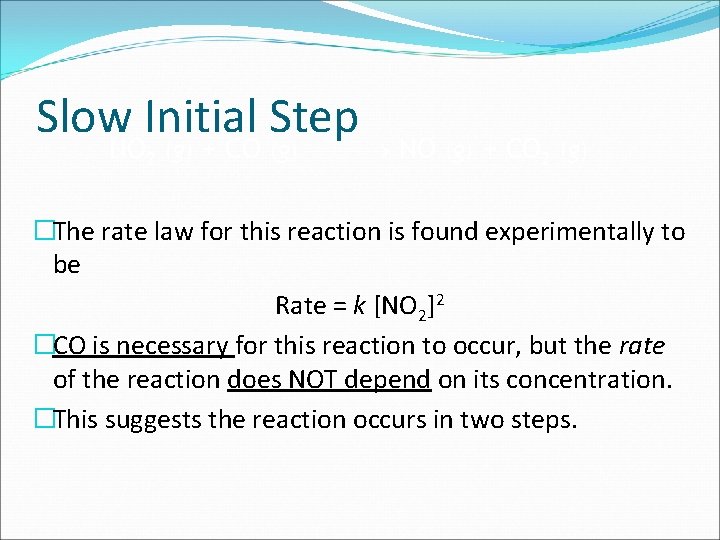
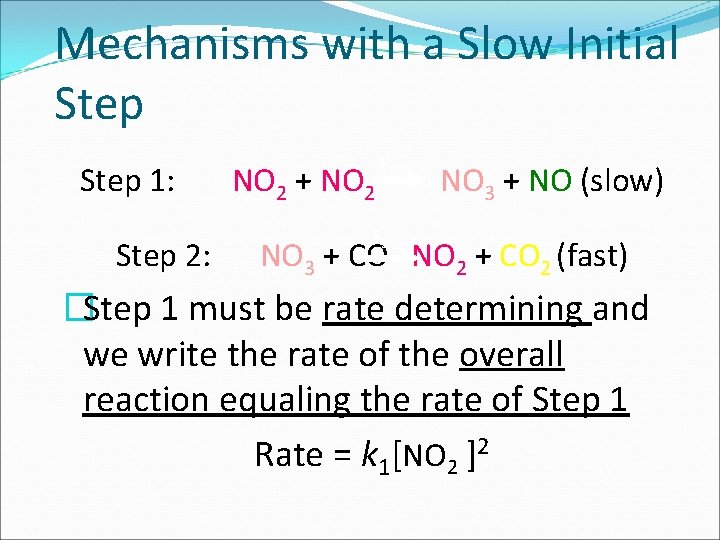
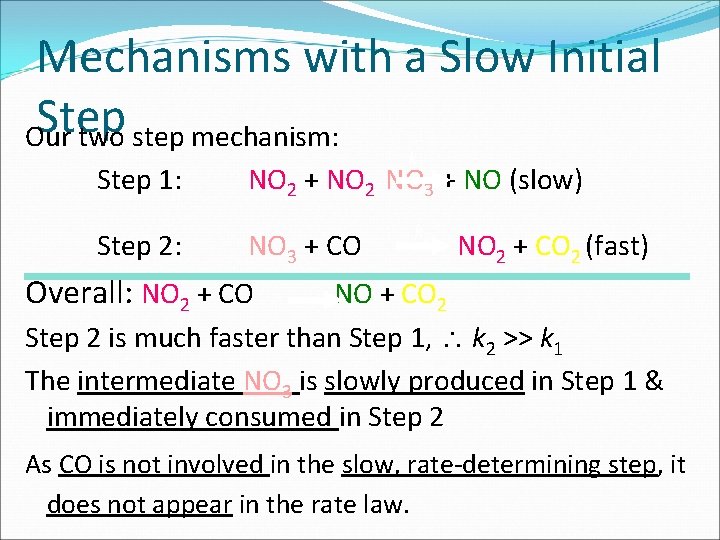
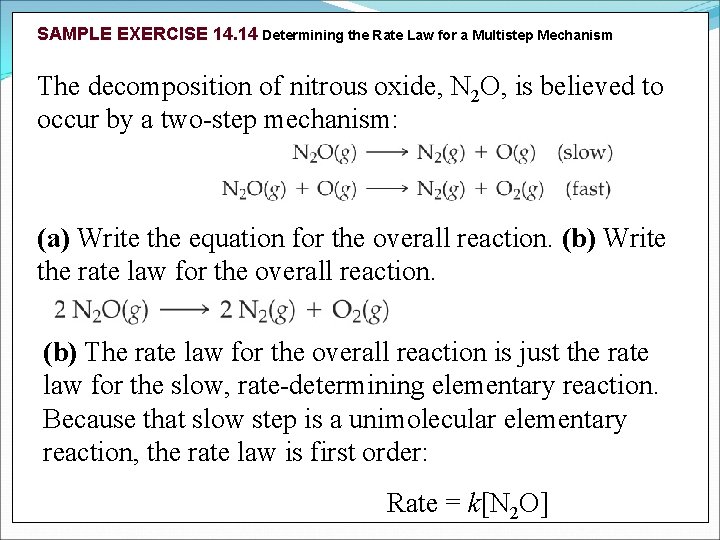
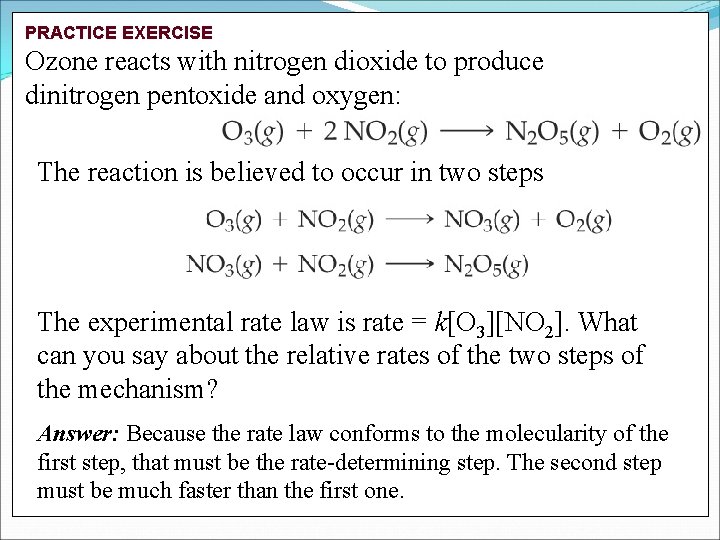
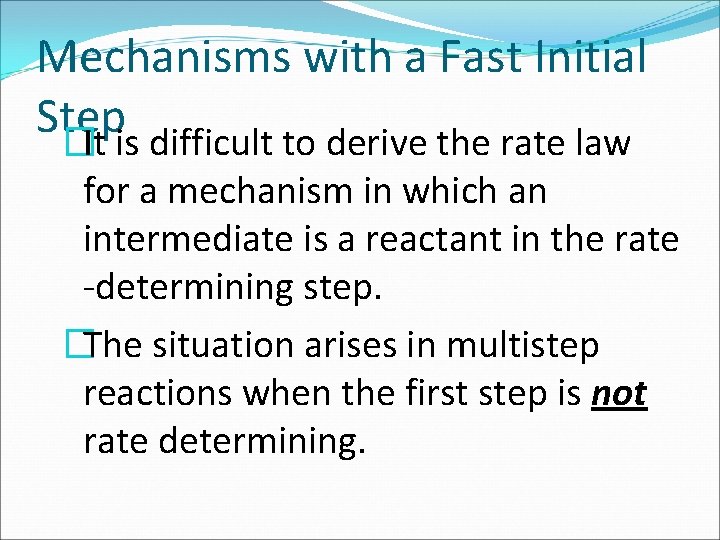
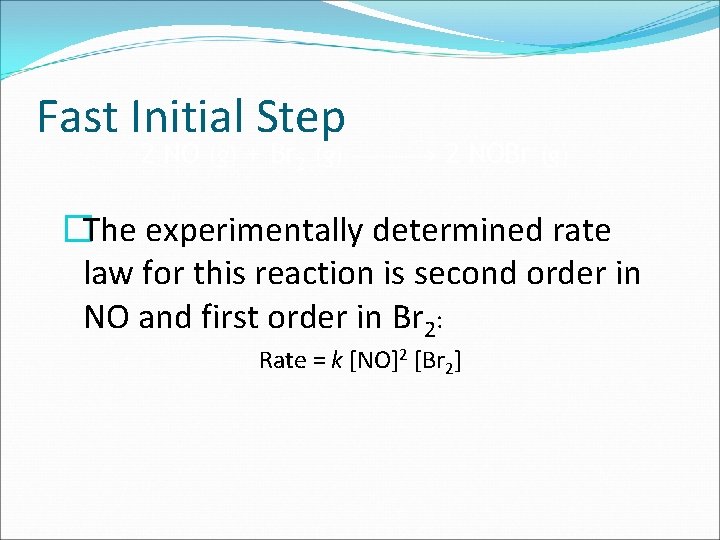
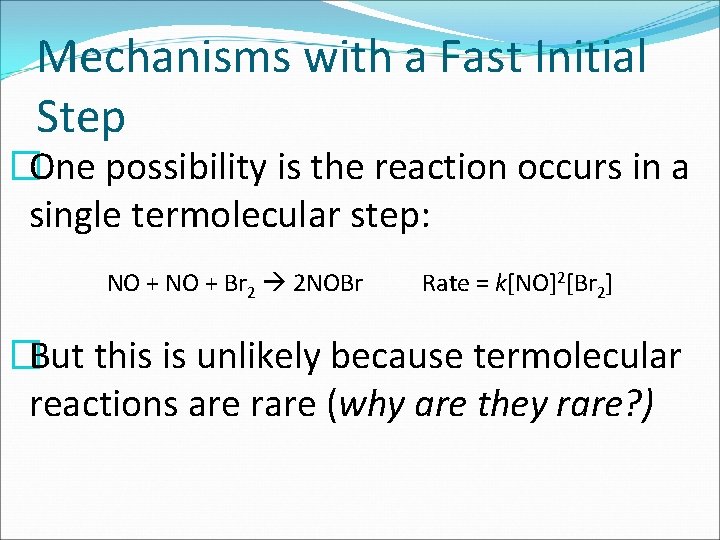
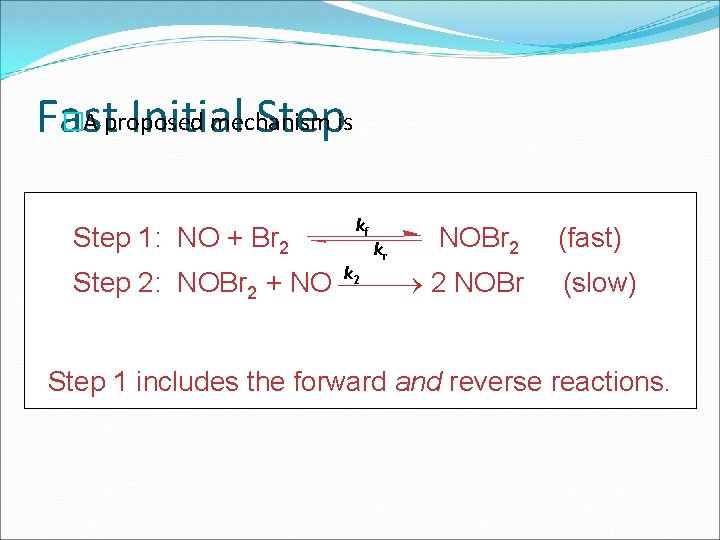
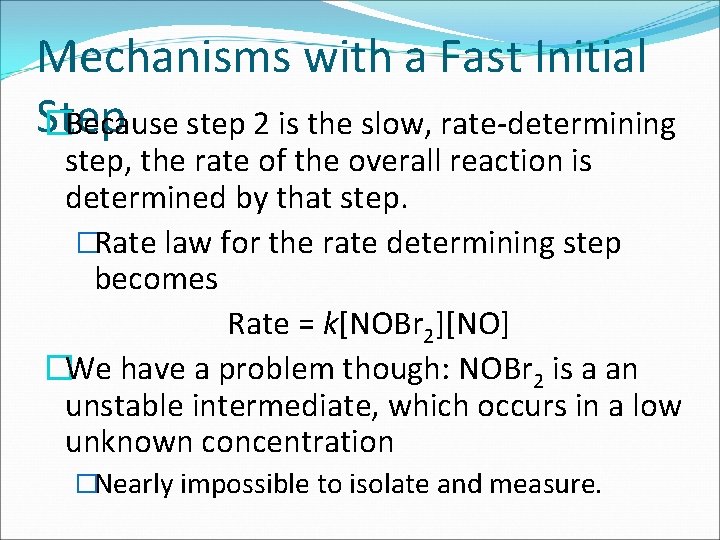
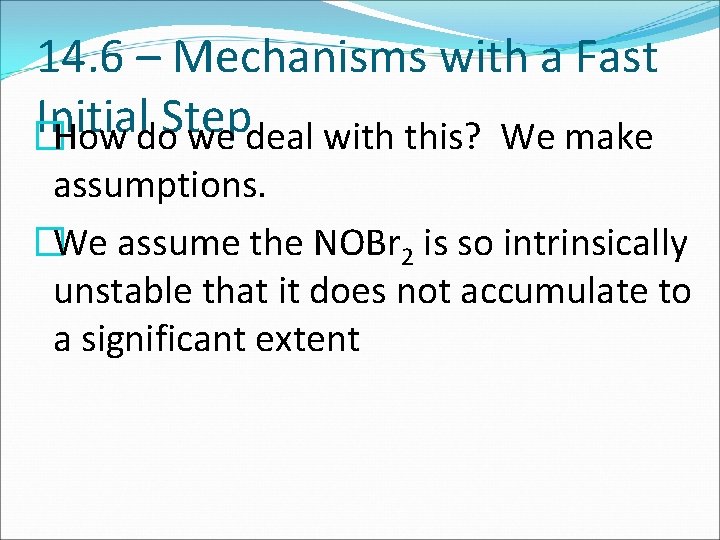
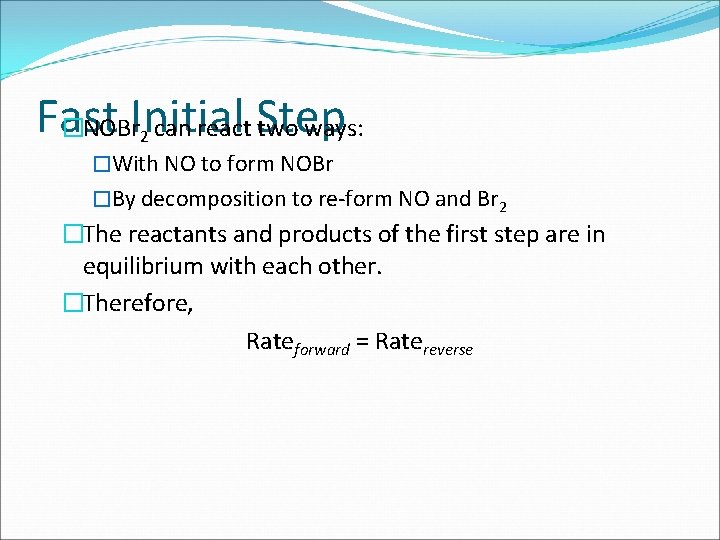
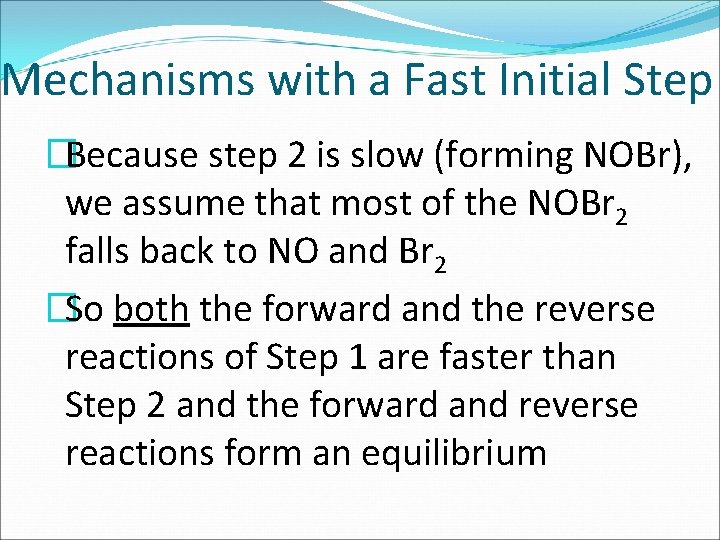
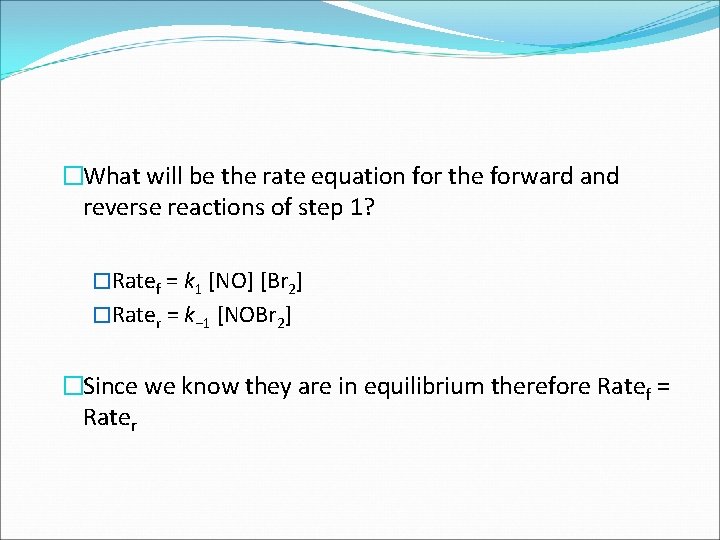
![Fast Initial Step �Because Ratef = Rater , k 1 [NO] [Br 2] = Fast Initial Step �Because Ratef = Rater , k 1 [NO] [Br 2] =](https://slidetodoc.com/presentation_image_h/1c9abc1fe8d10b8ee4aadf33e9ca8558/image-136.jpg)
![Substituting this Step expression for [NOBr ] in the rate law Fast Initial for Substituting this Step expression for [NOBr ] in the rate law Fast Initial for](https://slidetodoc.com/presentation_image_h/1c9abc1fe8d10b8ee4aadf33e9ca8558/image-137.jpg)
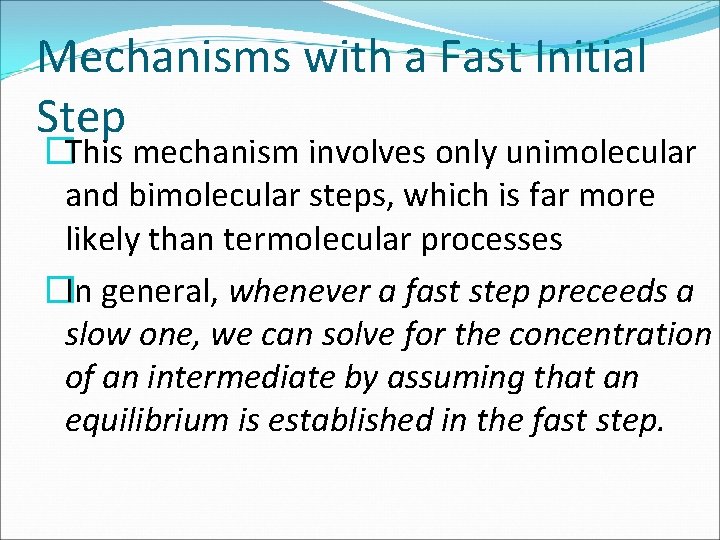
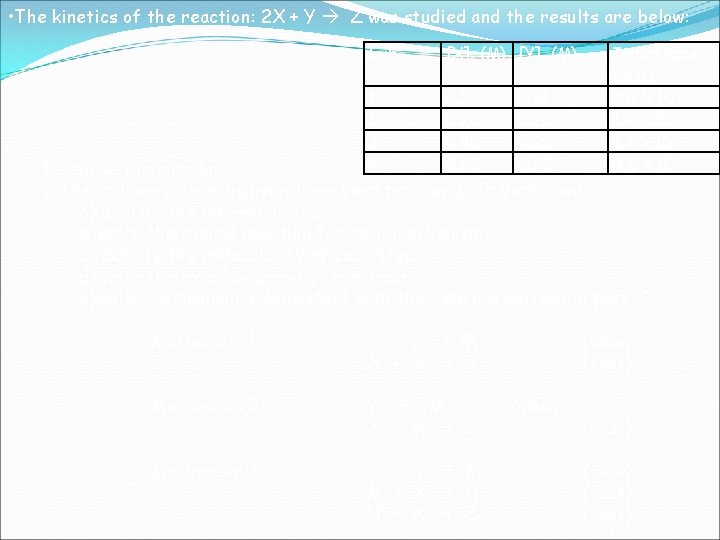
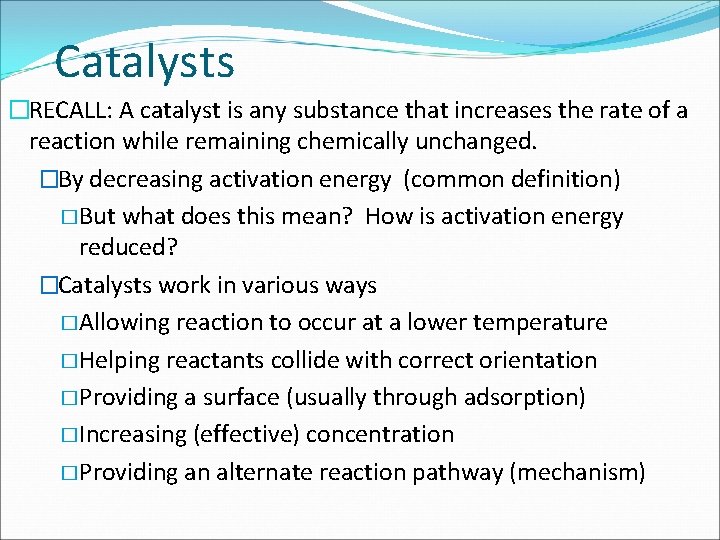
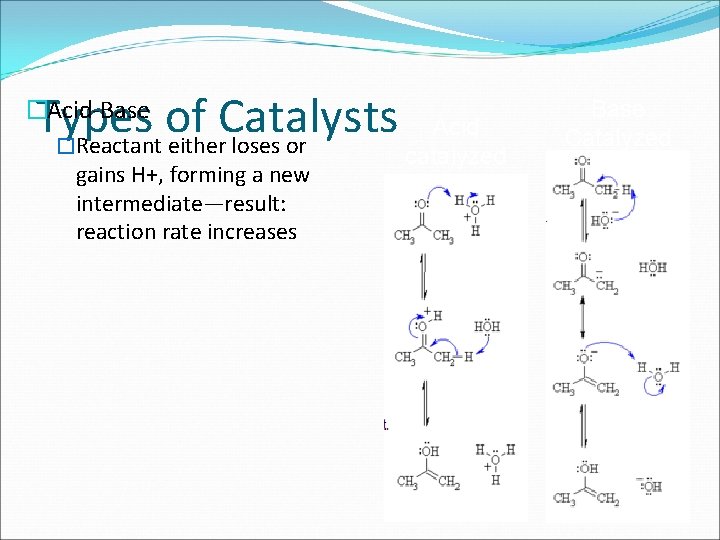
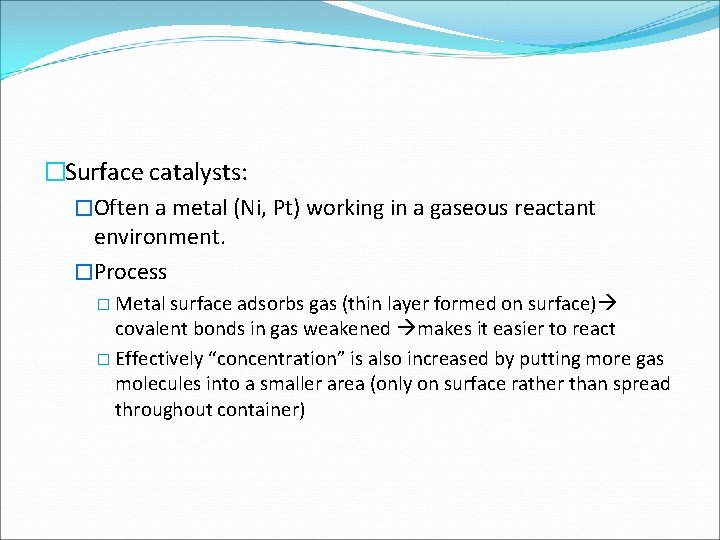
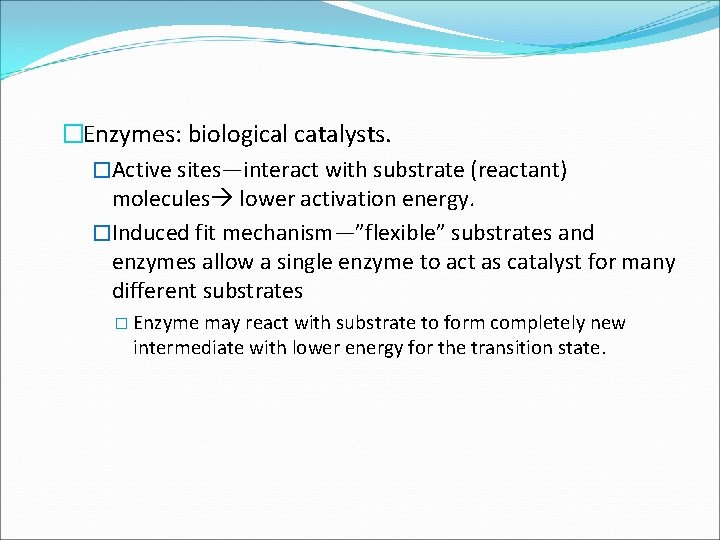
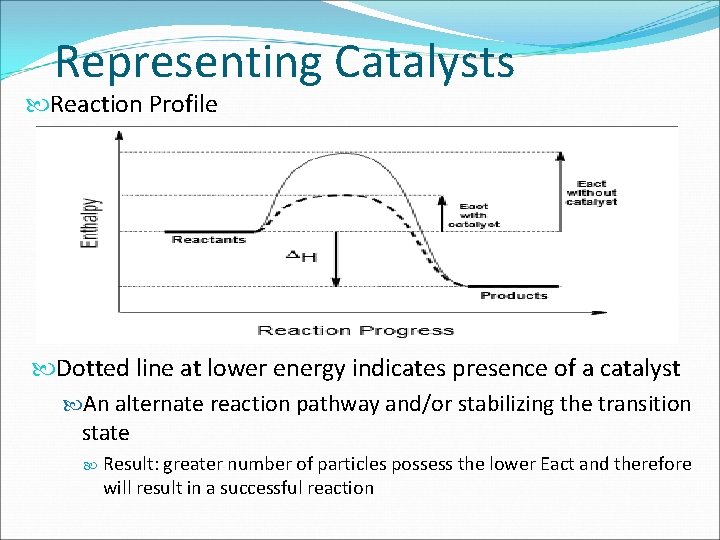
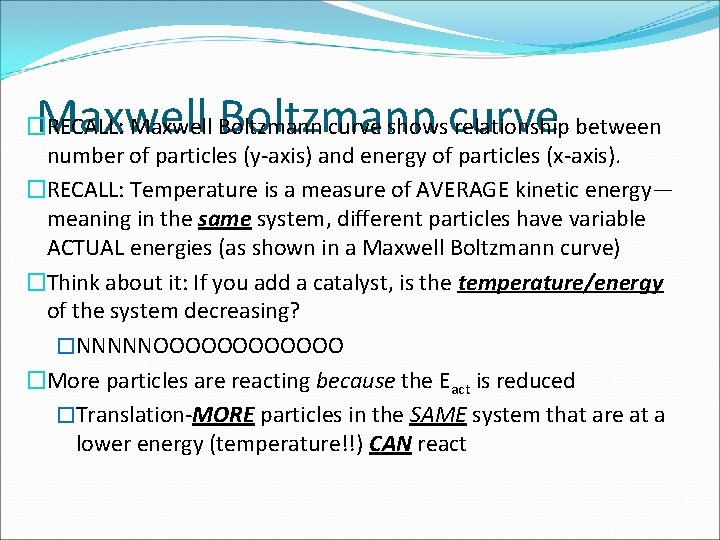
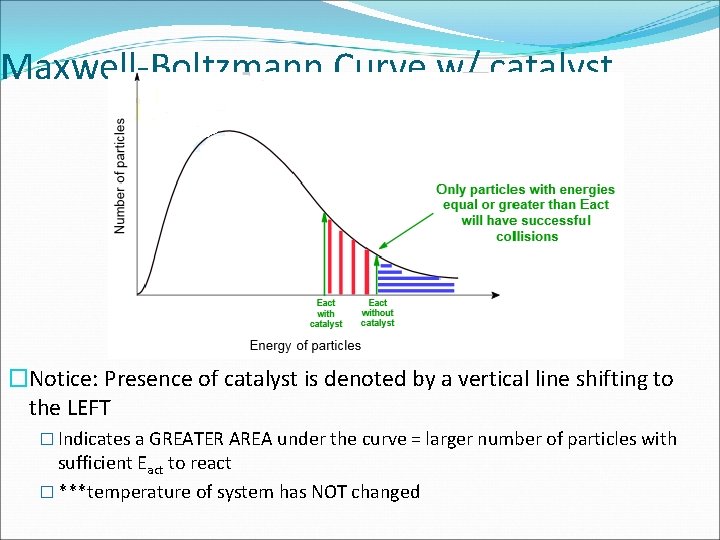
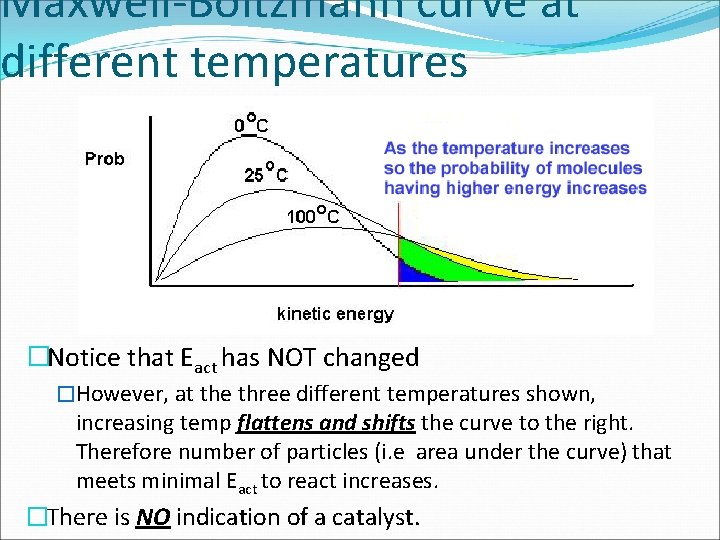
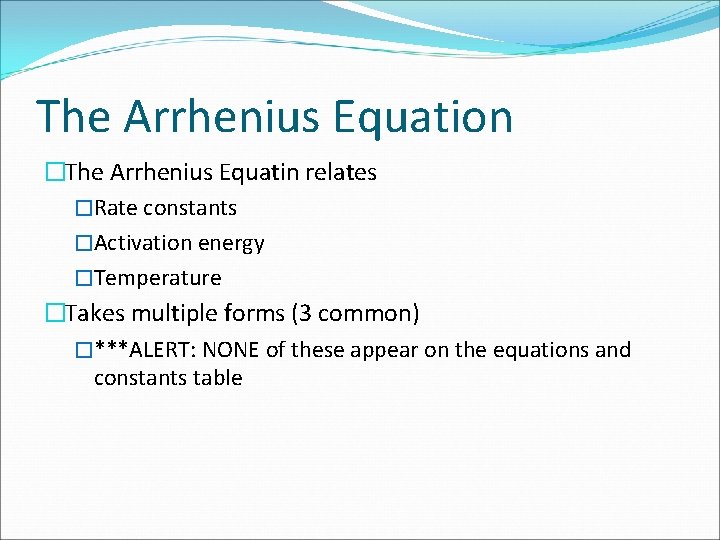
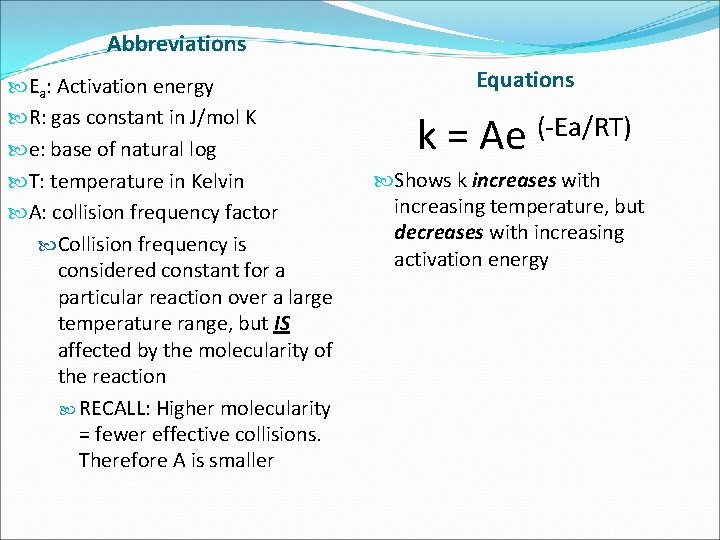
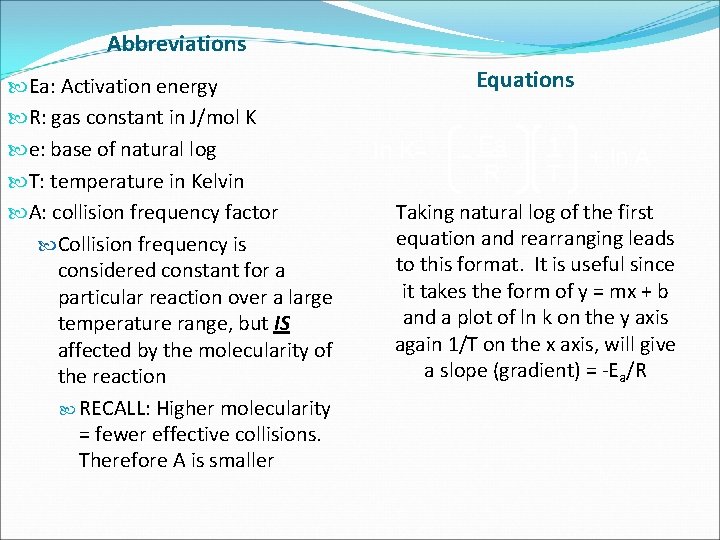
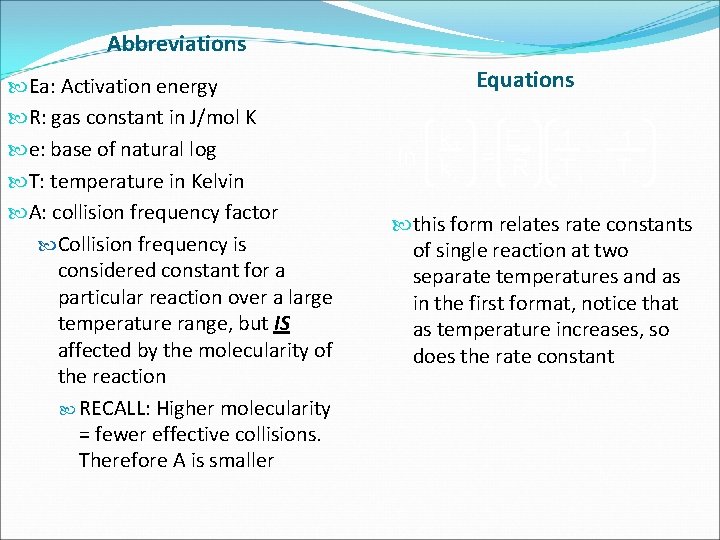
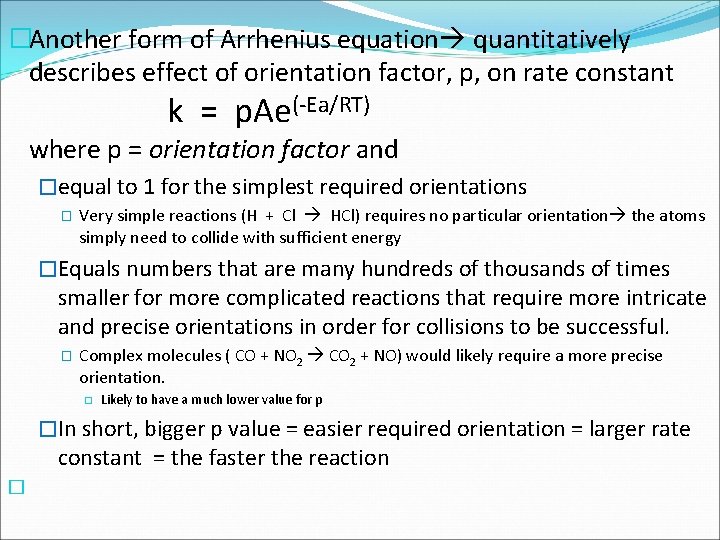
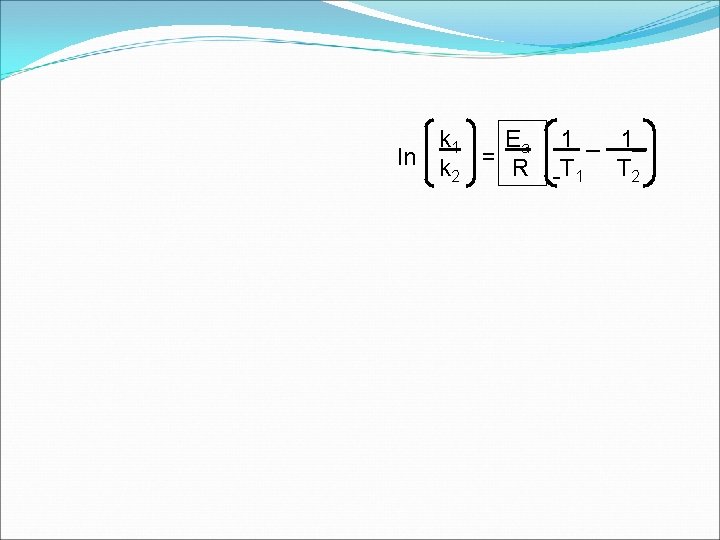
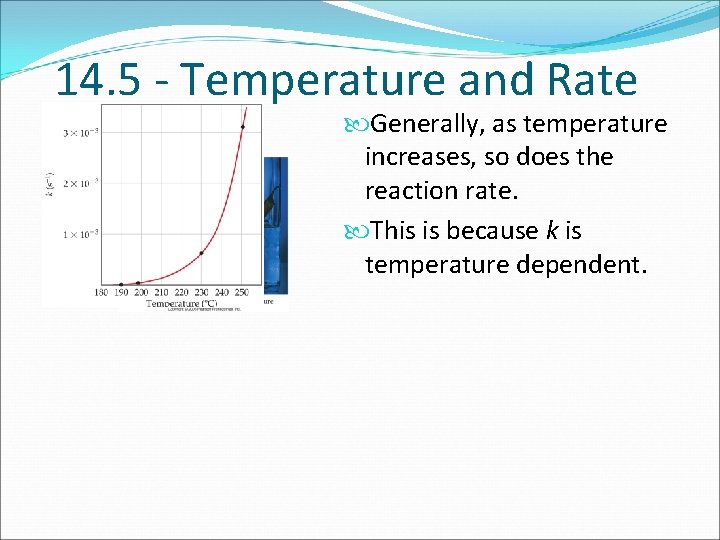
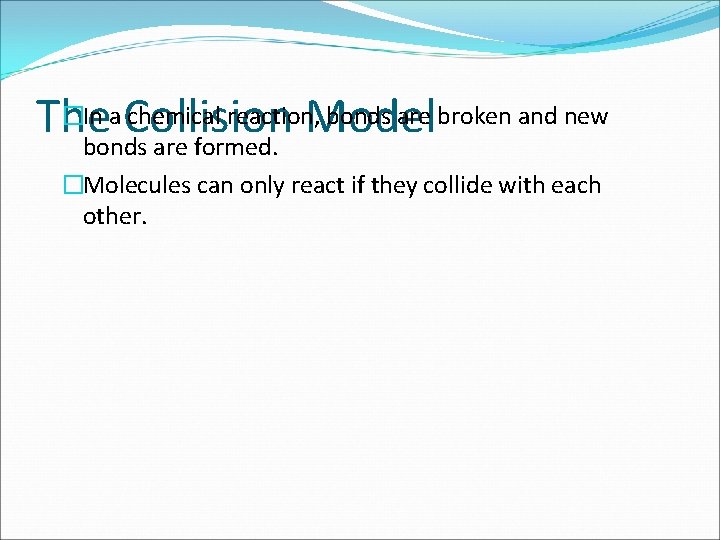
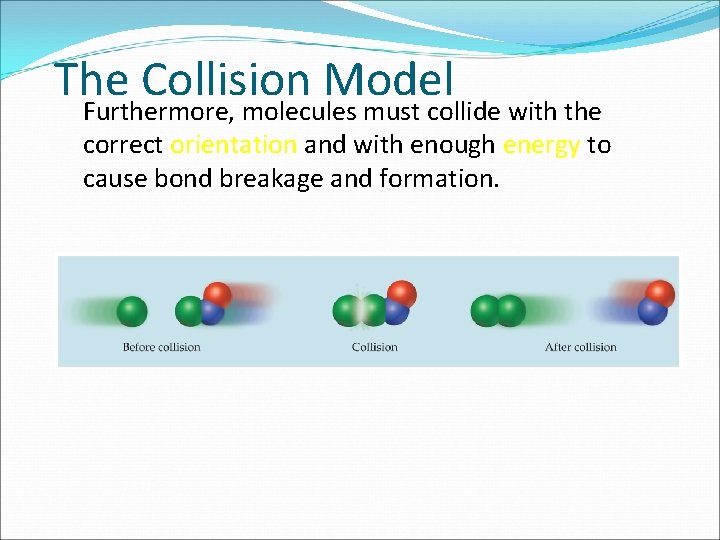
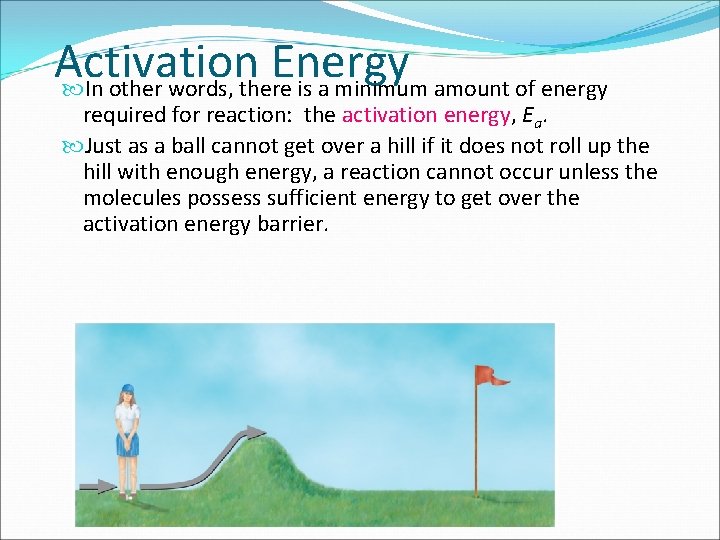
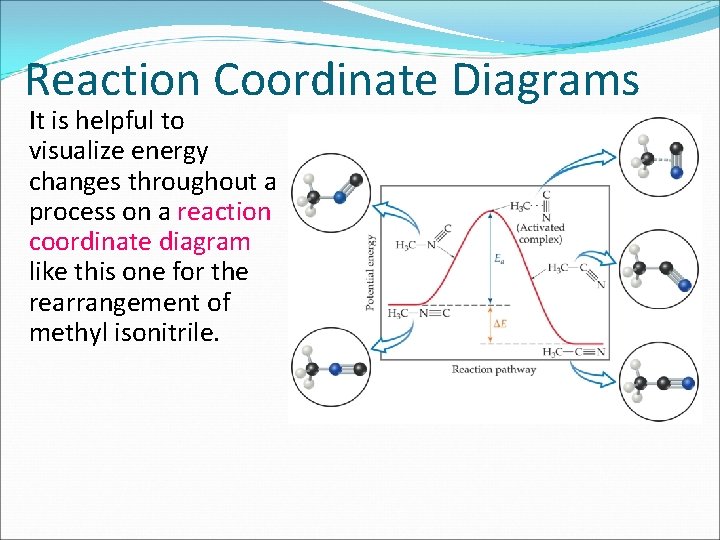
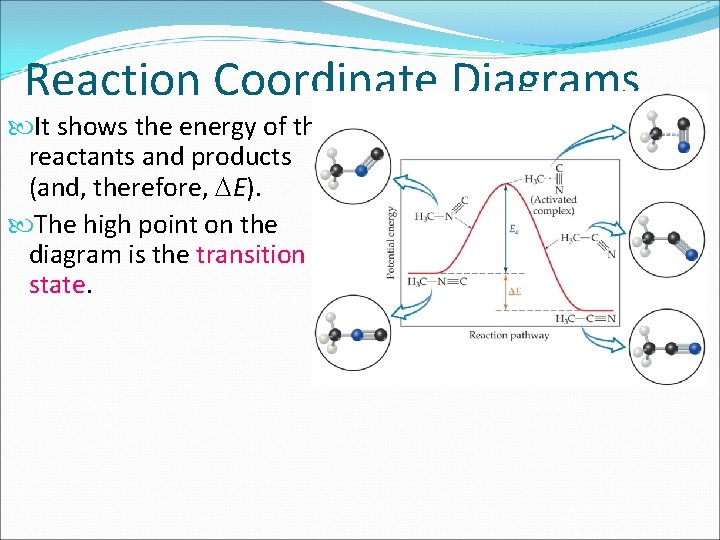
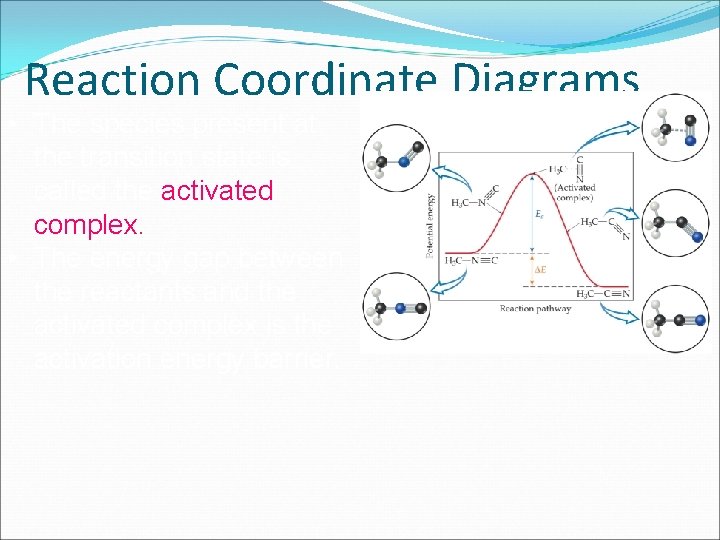
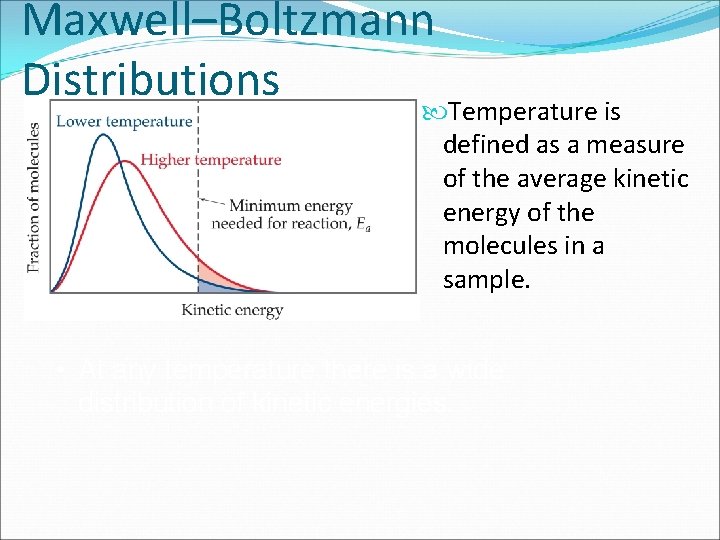
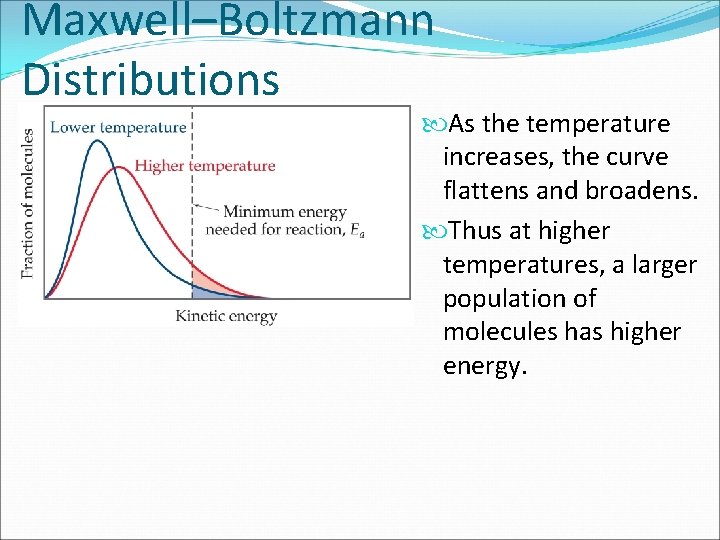
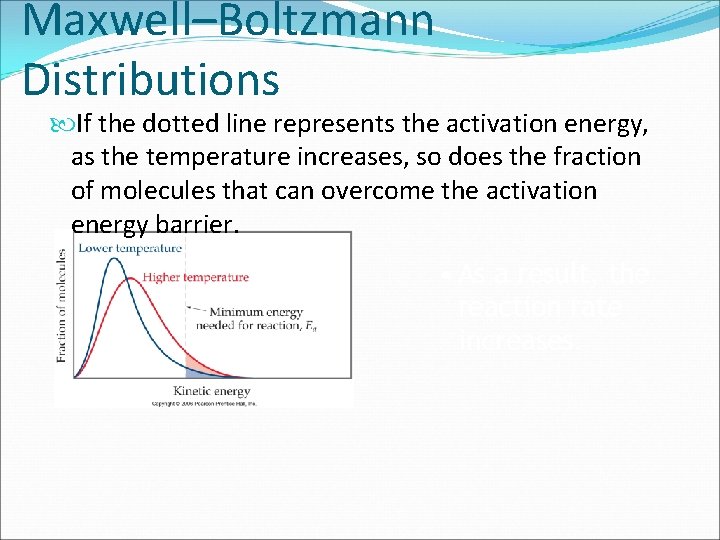
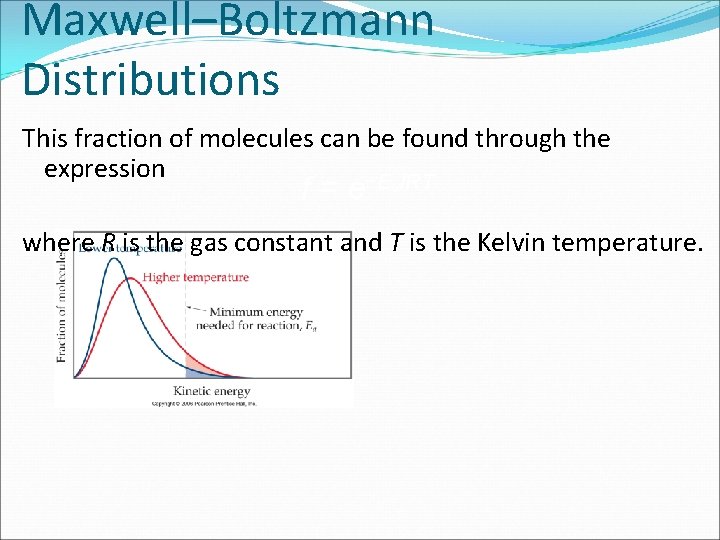
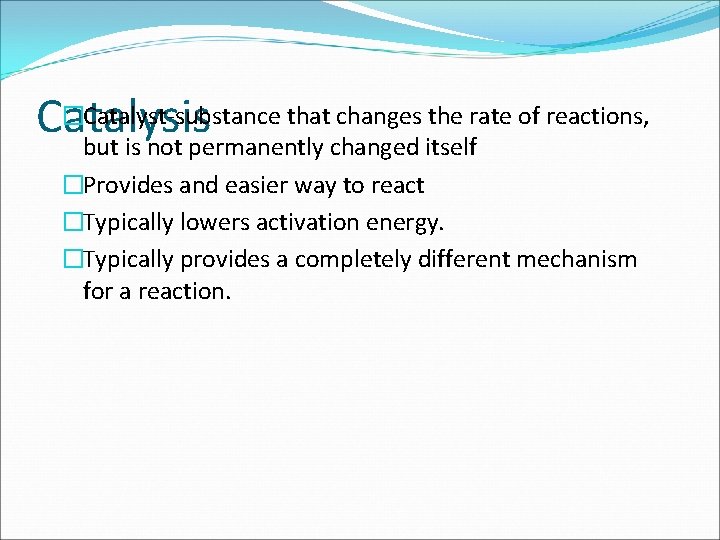
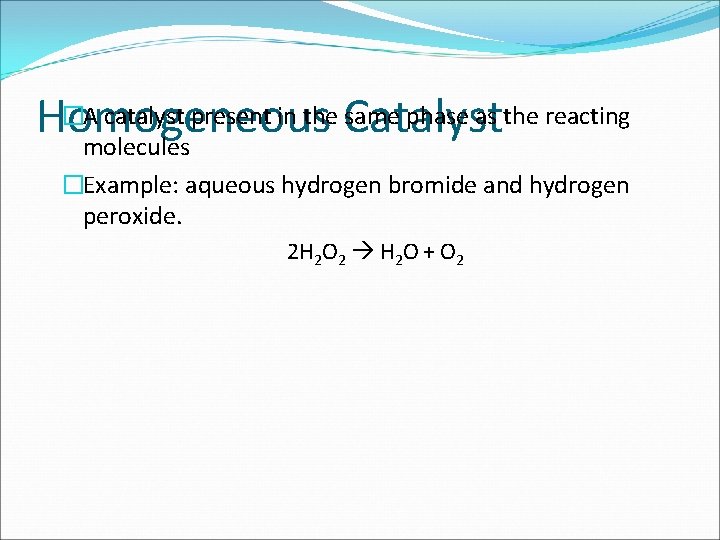
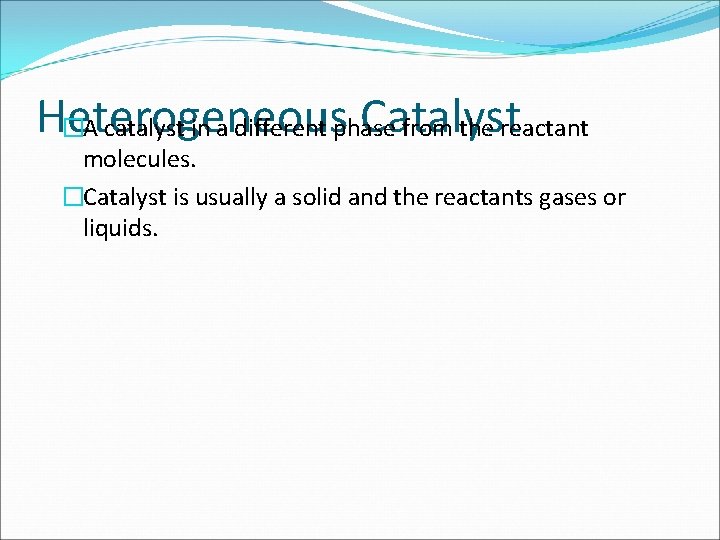
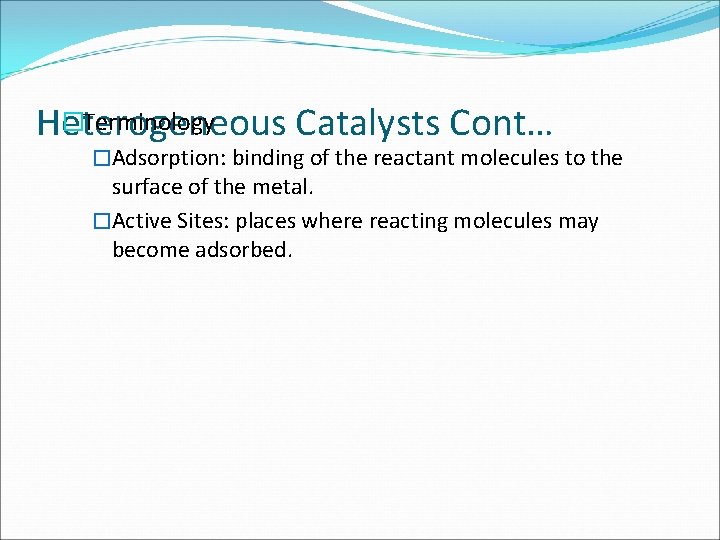
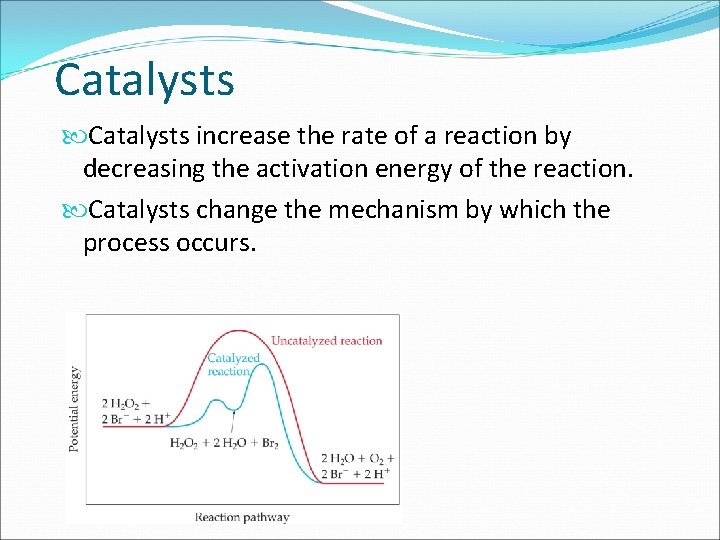
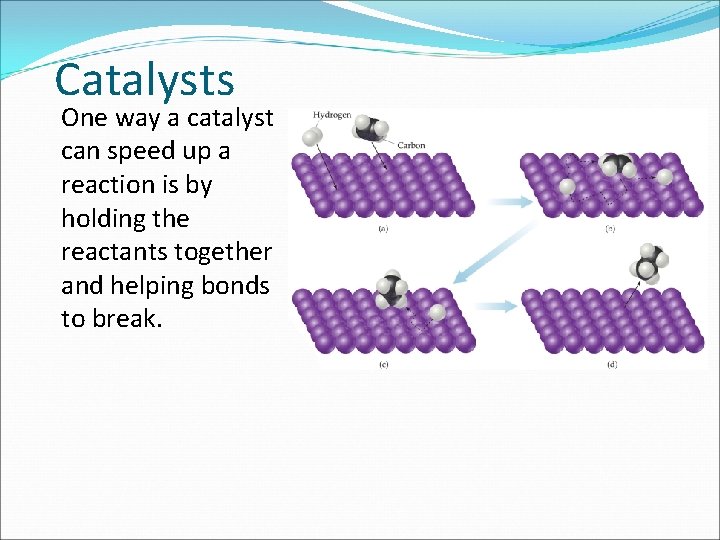
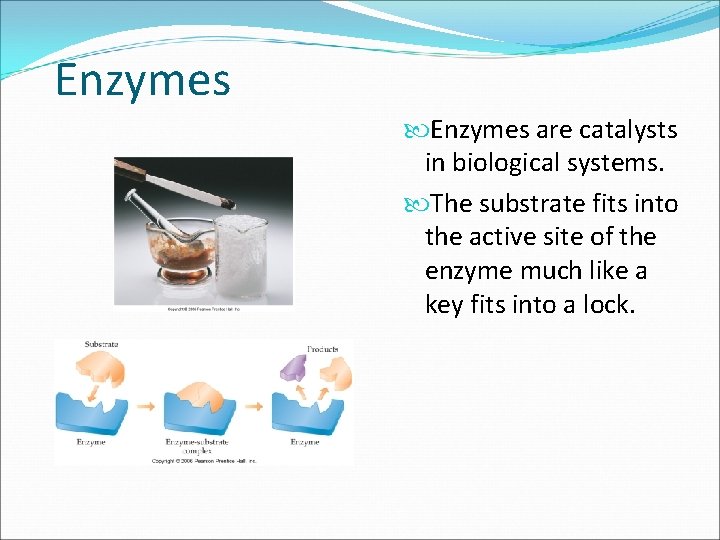
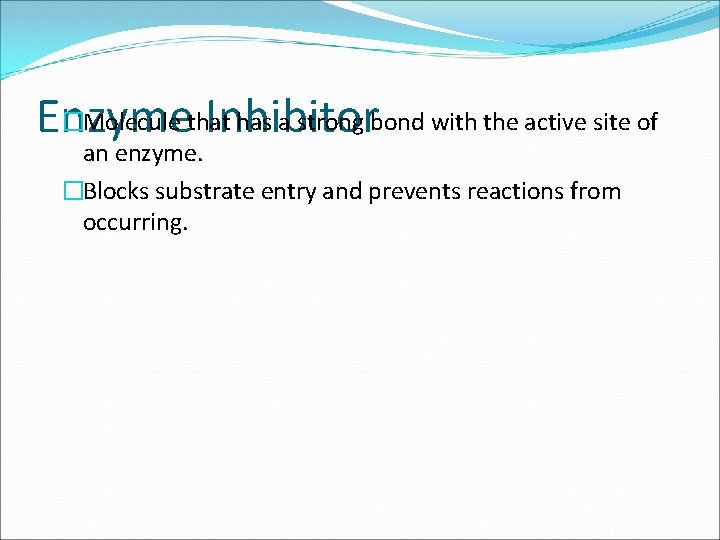
- Slides: 172
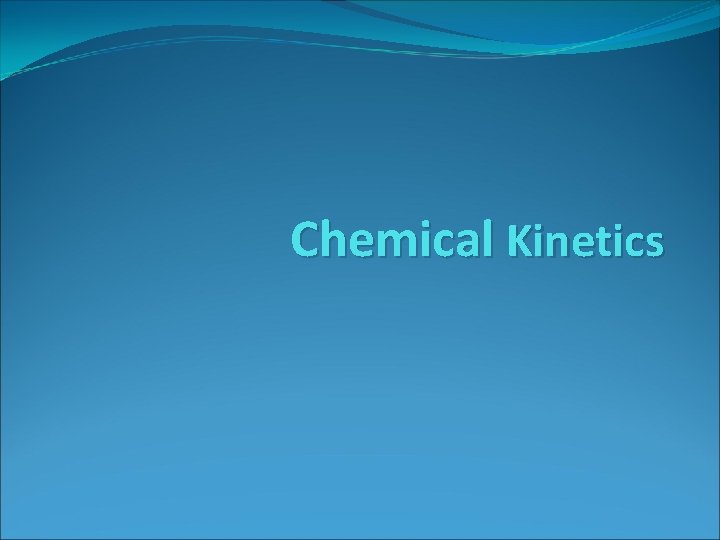
Chemical Kinetics
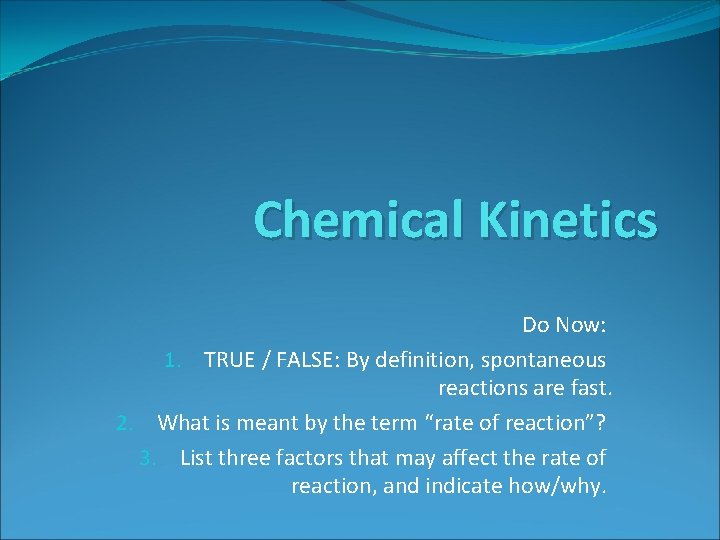
Chemical Kinetics Do Now: 1. TRUE / FALSE: By definition, spontaneous reactions are fast. 2. What is meant by the term “rate of reaction”? 3. List three factors that may affect the rate of reaction, and indicate how/why.
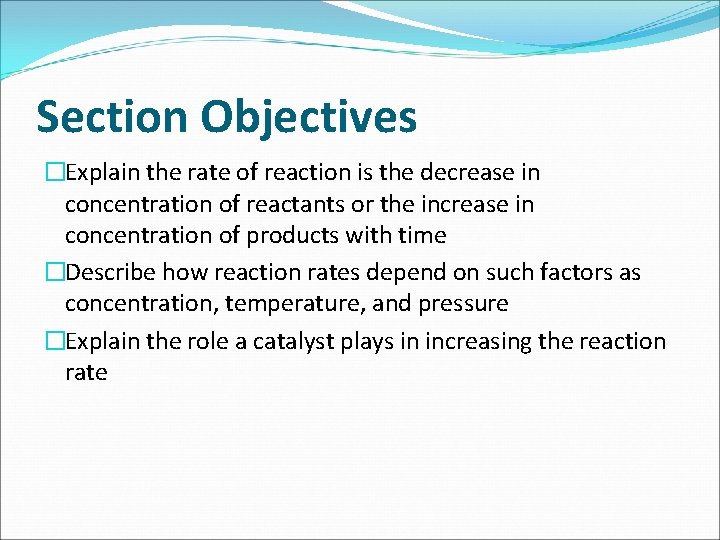
Section Objectives �Explain the rate of reaction is the decrease in concentration of reactants or the increase in concentration of products with time �Describe how reaction rates depend on such factors as concentration, temperature, and pressure �Explain the role a catalyst plays in increasing the reaction rate
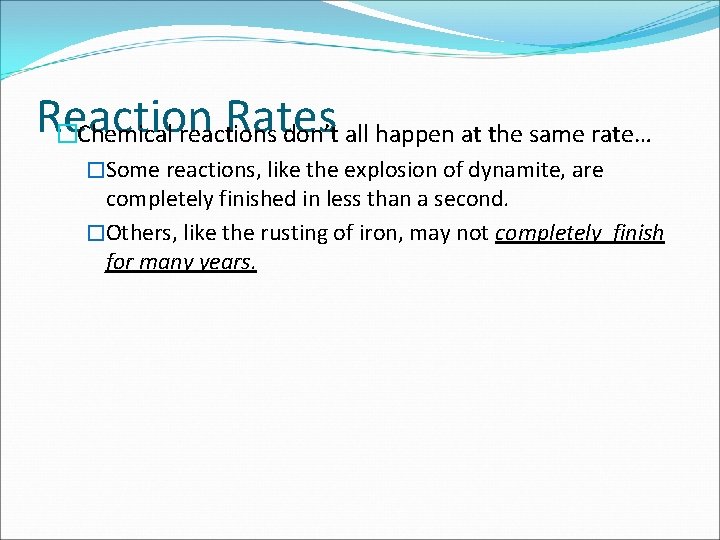
Reaction Rates �Chemical reactions don’t all happen at the same rate… �Some reactions, like the explosion of dynamite, are completely finished in less than a second. �Others, like the rusting of iron, may not completely finish for many years.
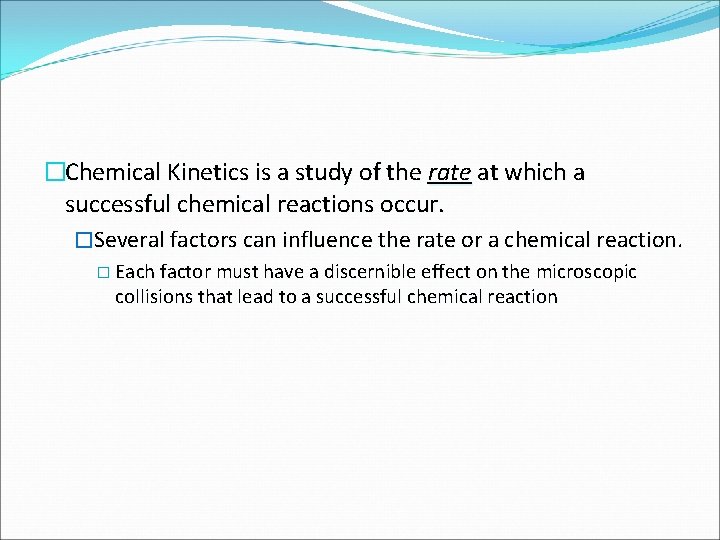
�Chemical Kinetics is a study of the rate at which a successful chemical reactions occur. �Several factors can influence the rate or a chemical reaction. � Each factor must have a discernible effect on the microscopic collisions that lead to a successful chemical reaction
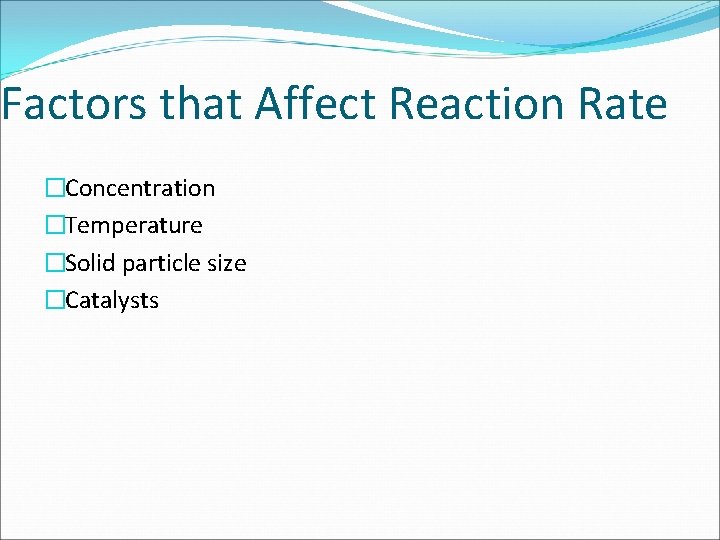
Factors that Affect Reaction Rate �Concentration �Temperature �Solid particle size �Catalysts
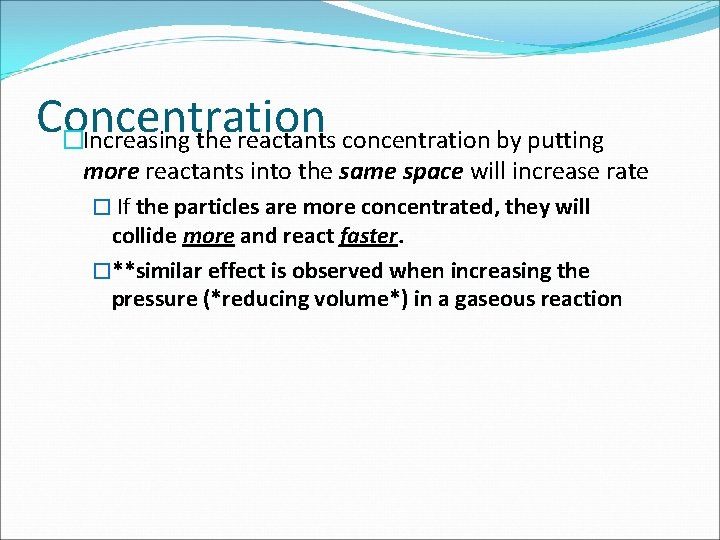
Concentration �Increasing the reactants concentration by putting more reactants into the same space will increase rate � If the particles are more concentrated, they will collide more and react faster. �**similar effect is observed when increasing the pressure (*reducing volume*) in a gaseous reaction
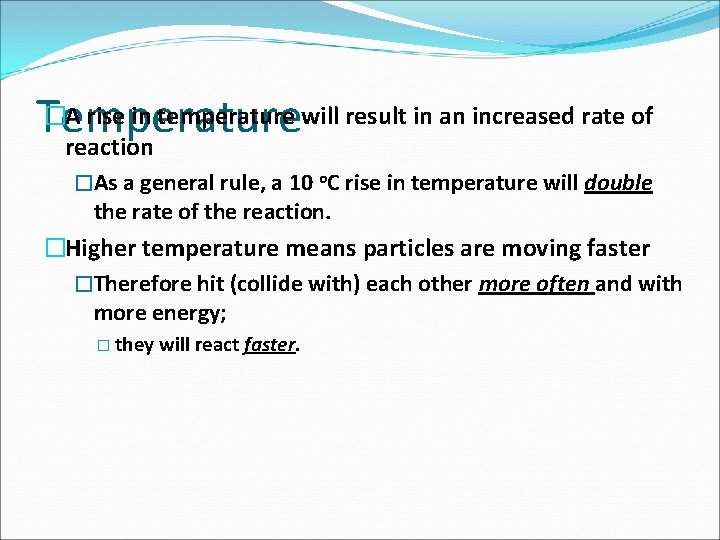
�A rise in temperature will result in an increased rate of Temperature reaction �As a general rule, a 10 o. C rise in temperature will double the rate of the reaction. �Higher temperature means particles are moving faster �Therefore hit (collide with) each other more often and with more energy; � they will react faster.
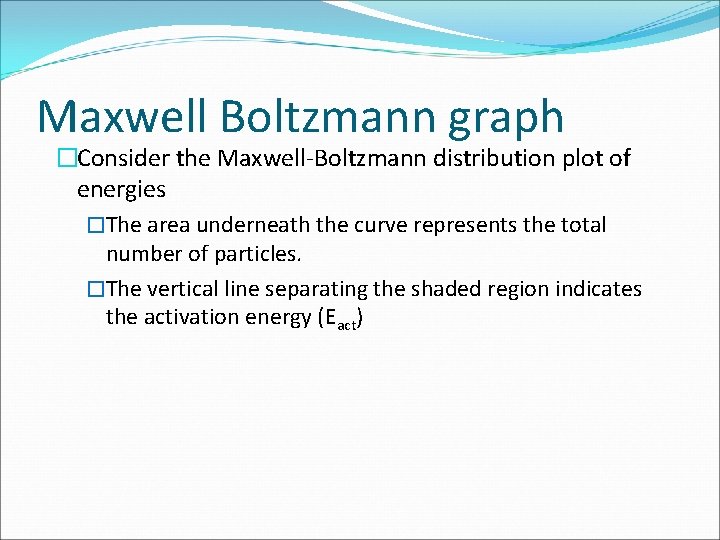
Maxwell Boltzmann graph �Consider the Maxwell-Boltzmann distribution plot of energies �The area underneath the curve represents the total number of particles. �The vertical line separating the shaded region indicates the activation energy (Eact)
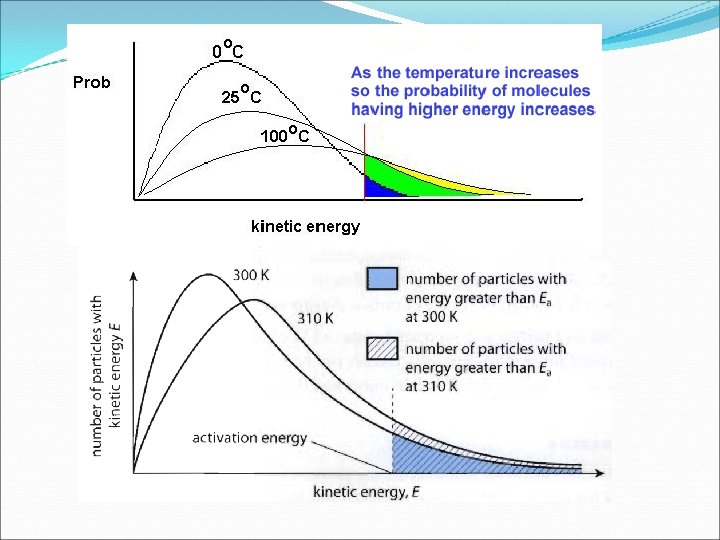
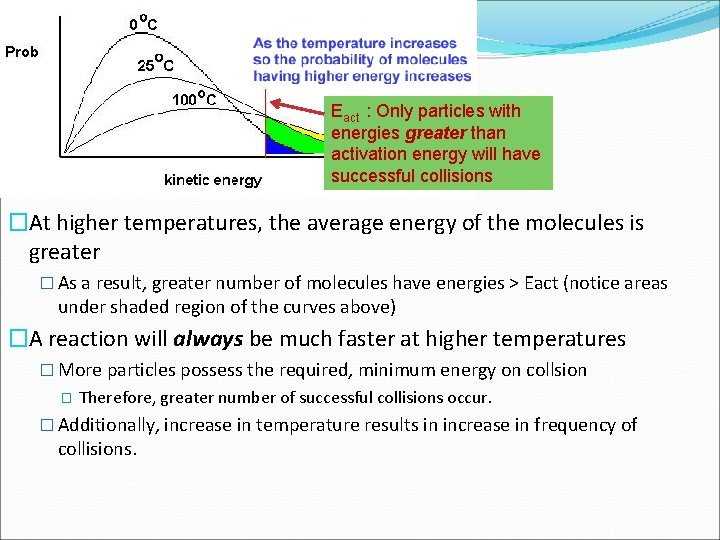
Eact : Only particles with energies greater than activation energy will have successful collisions �At higher temperatures, the average energy of the molecules is greater � As a result, greater number of molecules have energies > Eact (notice areas under shaded region of the curves above) �A reaction will always be much faster at higher temperatures � More particles possess the required, minimum energy on collsion � Therefore, greater number of successful collisions occur. � Additionally, increase in temperature results in increase in frequency of collisions.
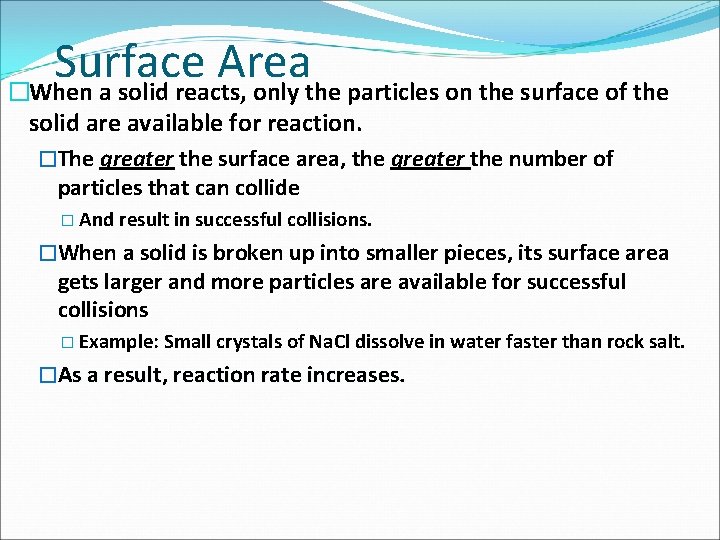
Surface Area �When a solid reacts, only the particles on the surface of the solid are available for reaction. �The greater the surface area, the greater the number of particles that can collide � And result in successful collisions. �When a solid is broken up into smaller pieces, its surface area gets larger and more particles are available for successful collisions � Example: Small crystals of Na. Cl dissolve in water faster than rock salt. �As a result, reaction rate increases.
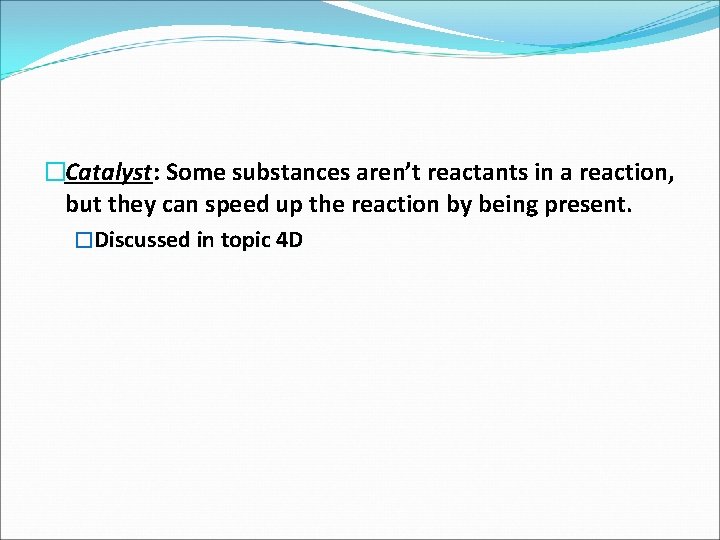
�Catalyst: Some substances aren’t reactants in a reaction, but they can speed up the reaction by being present. �Discussed in topic 4 D
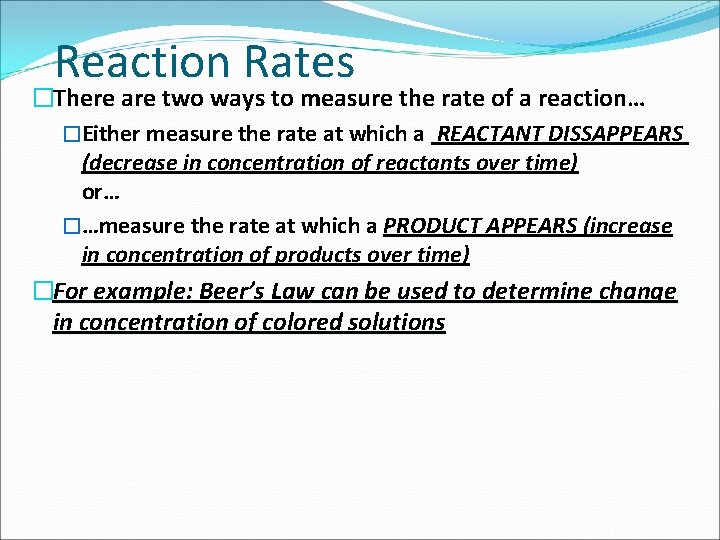
Reaction Rates �There are two ways to measure the rate of a reaction… �Either measure the rate at which a REACTANT DISSAPPEARS (decrease in concentration of reactants over time) or… �…measure the rate at which a PRODUCT APPEARS (increase in concentration of products over time) �For example: Beer’s Law can be used to determine change in concentration of colored solutions
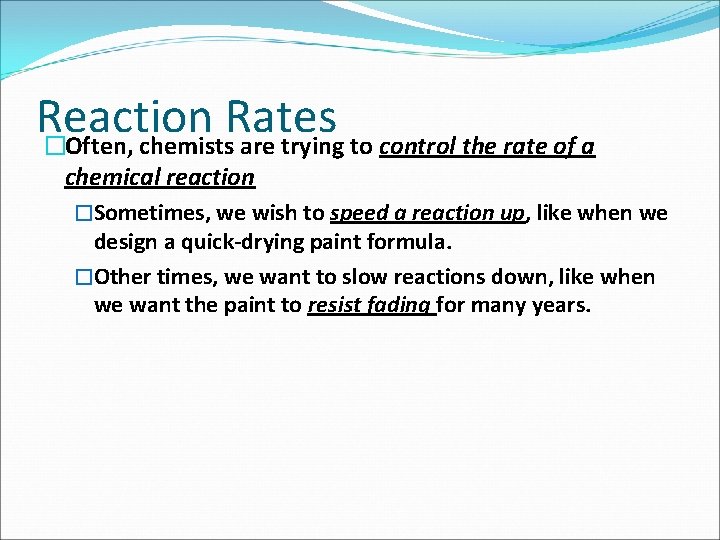
Reaction Rates �Often, chemists are trying to control the rate of a chemical reaction �Sometimes, we wish to speed a reaction up, like when we design a quick-drying paint formula. �Other times, we want to slow reactions down, like when we want the paint to resist fading for many years.
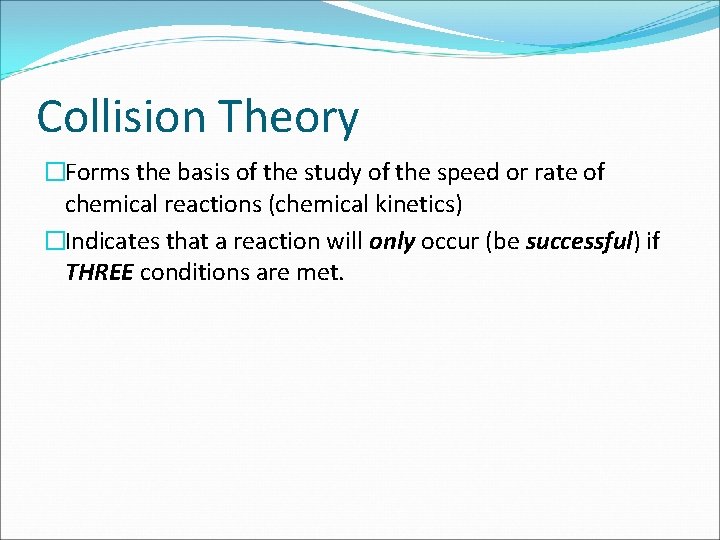
Collision Theory �Forms the basis of the study of the speed or rate of chemical reactions (chemical kinetics) �Indicates that a reaction will only occur (be successful) if THREE conditions are met.
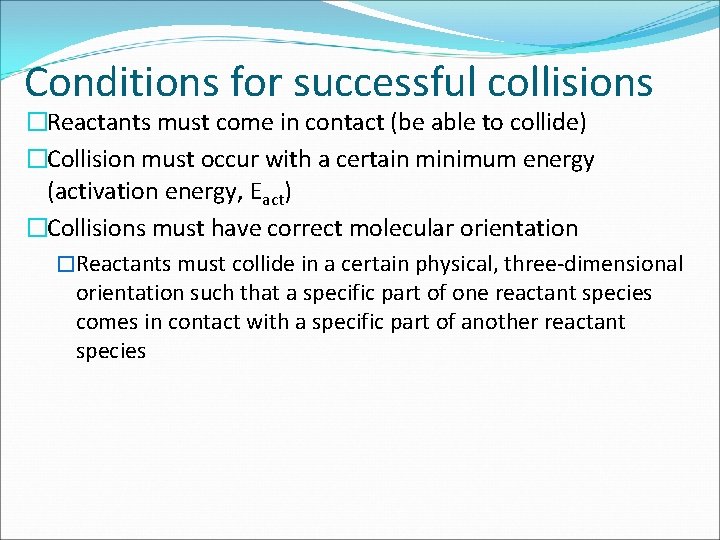
Conditions for successful collisions �Reactants must come in contact (be able to collide) �Collision must occur with a certain minimum energy (activation energy, Eact) �Collisions must have correct molecular orientation �Reactants must collide in a certain physical, three-dimensional orientation such that a specific part of one reactant species comes in contact with a specific part of another reactant species
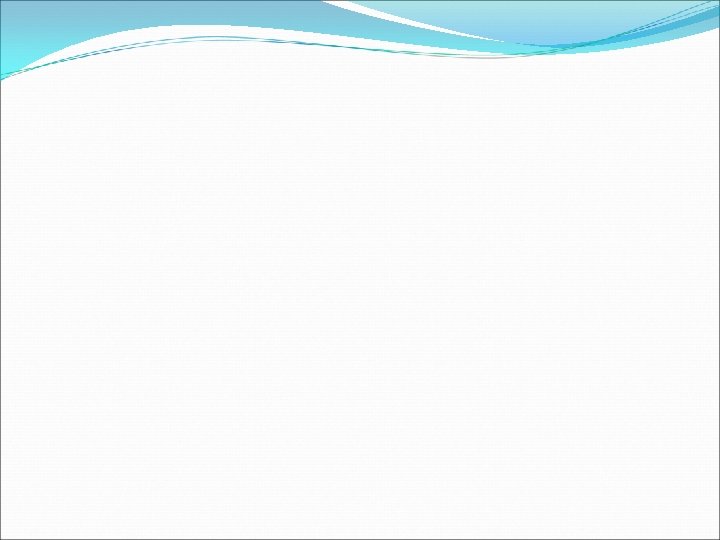
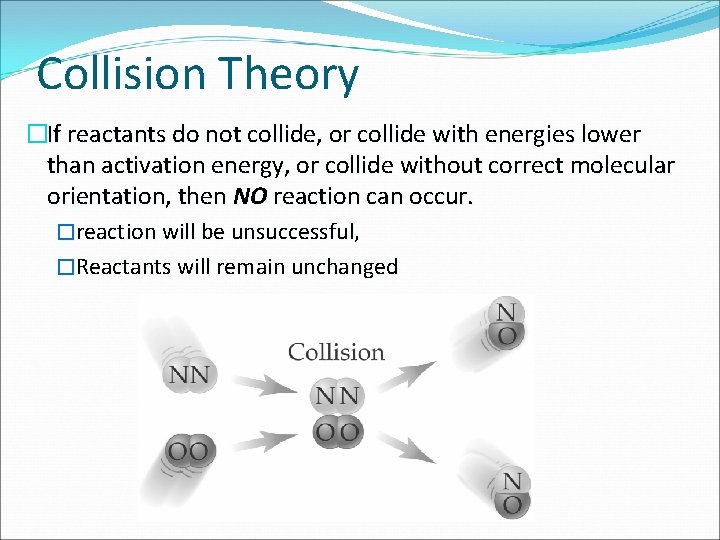
Collision Theory �If reactants do not collide, or collide with energies lower than activation energy, or collide without correct molecular orientation, then NO reaction can occur. �reaction will be unsuccessful, �Reactants will remain unchanged
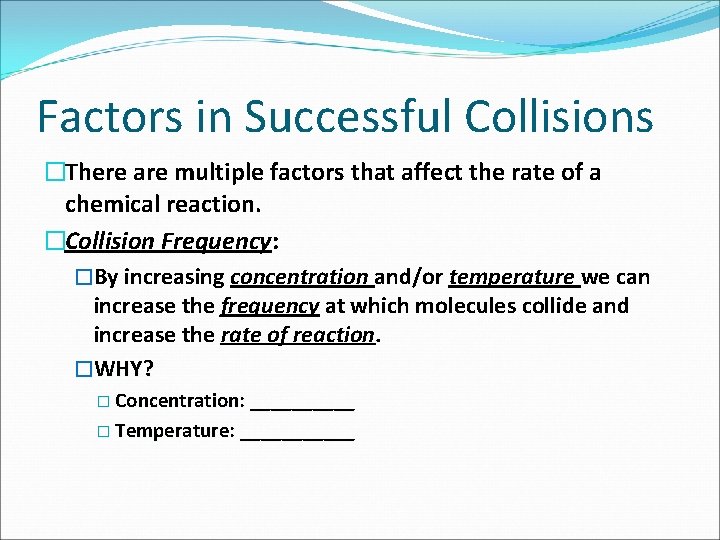
Factors in Successful Collisions �There are multiple factors that affect the rate of a chemical reaction. �Collision Frequency: �By increasing concentration and/or temperature we can increase the frequency at which molecules collide and increase the rate of reaction. �WHY? � Concentration: _____ � Temperature: ______
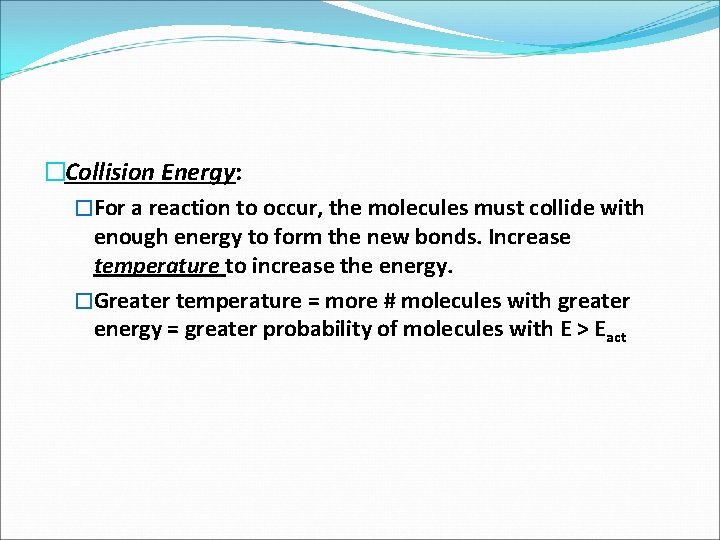
�Collision Energy: �For a reaction to occur, the molecules must collide with enough energy to form the new bonds. Increase temperature to increase the energy. �Greater temperature = more # molecules with greater energy = greater probability of molecules with E > Eact
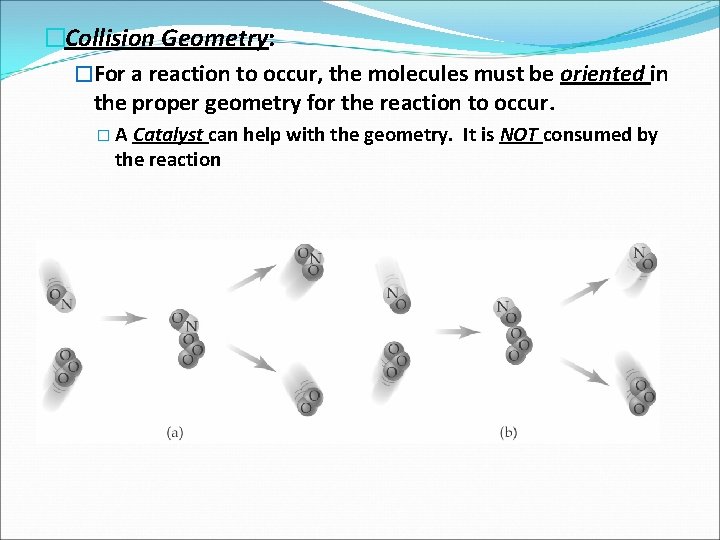
�Collision Geometry: �For a reaction to occur, the molecules must be oriented in the proper geometry for the reaction to occur. �A Catalyst can help with the geometry. It is NOT consumed by the reaction
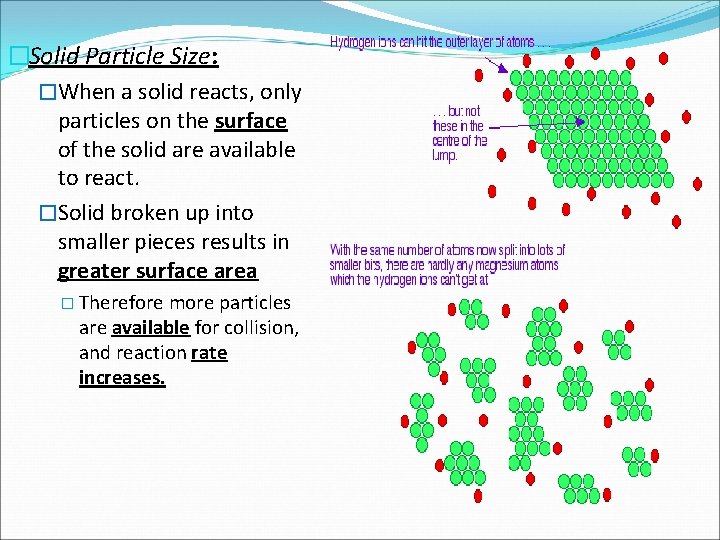
�Solid Particle Size: �When a solid reacts, only particles on the surface of the solid are available to react. �Solid broken up into smaller pieces results in greater surface area � Therefore more particles are available for collision, and reaction rate increases.
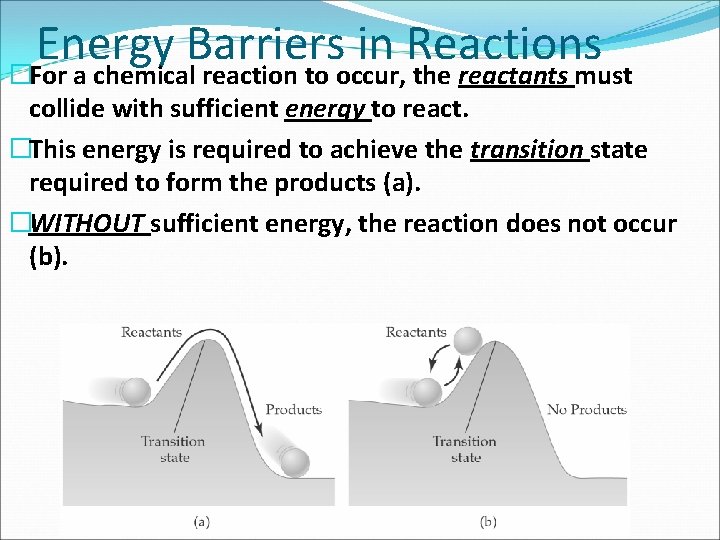
Energy Barriers in Reactions �For a chemical reaction to occur, the reactants must collide with sufficient energy to react. �This energy is required to achieve the transition state required to form the products (a). �WITHOUT sufficient energy, the reaction does not occur (b).
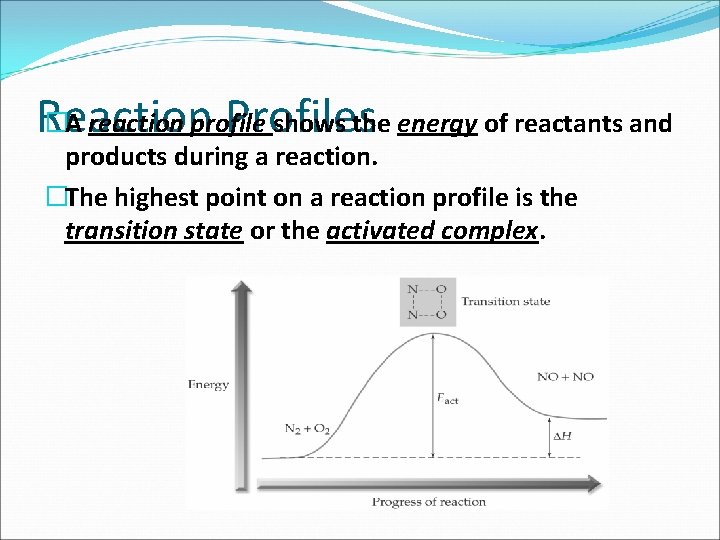
�A reaction profile shows the energy of reactants and Reaction Profiles products during a reaction. �The highest point on a reaction profile is the transition state or the activated complex.
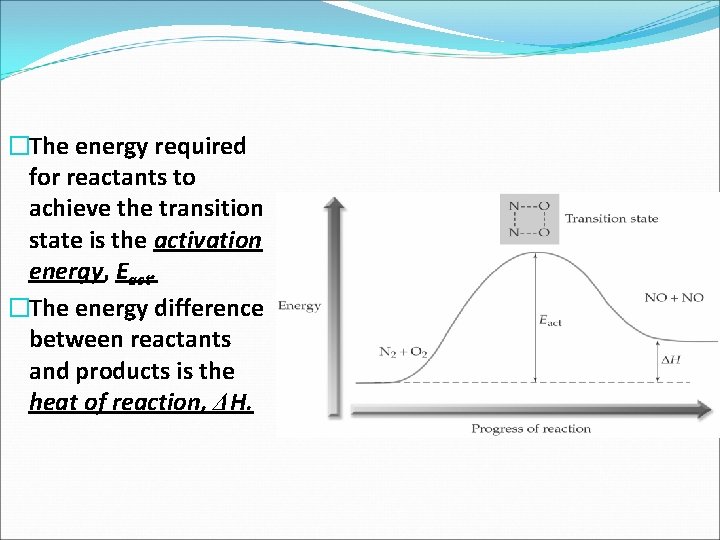
�The energy required for reactants to achieve the transition state is the activation energy, Eact. �The energy difference between reactants and products is the heat of reaction, ΔH.
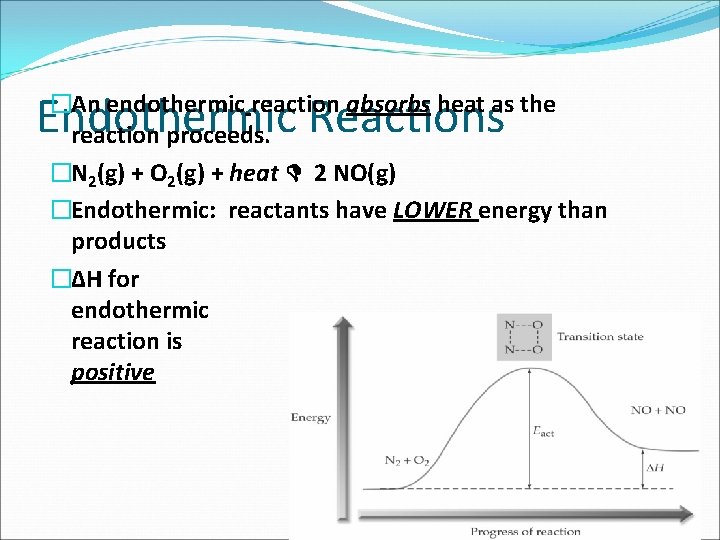
Endothermic Reactions �An endothermic reaction absorbs heat as the reaction proceeds. �N 2(g) + O 2(g) + heat 2 NO(g) �Endothermic: reactants have LOWER energy than products �ΔH for endothermic reaction is positive
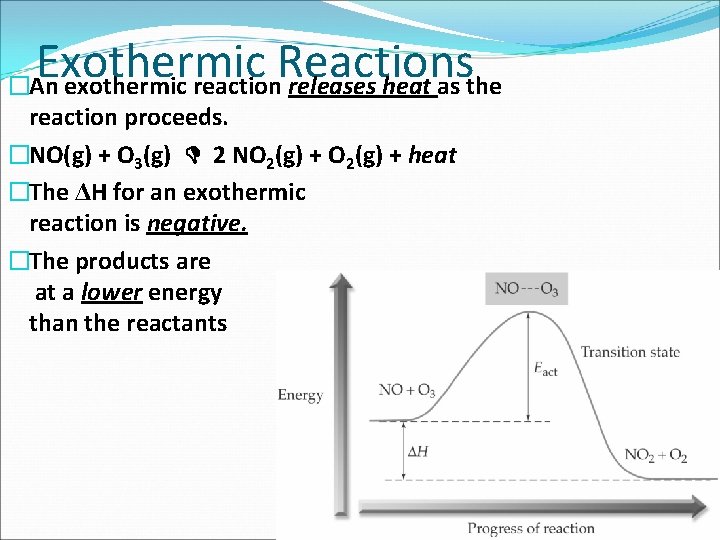
Exothermic Reactions �An exothermic reaction releases heat as the reaction proceeds. �NO(g) + O 3(g) 2 NO 2(g) + heat �The ΔH for an exothermic reaction is negative. �The products are at a lower energy than the reactants
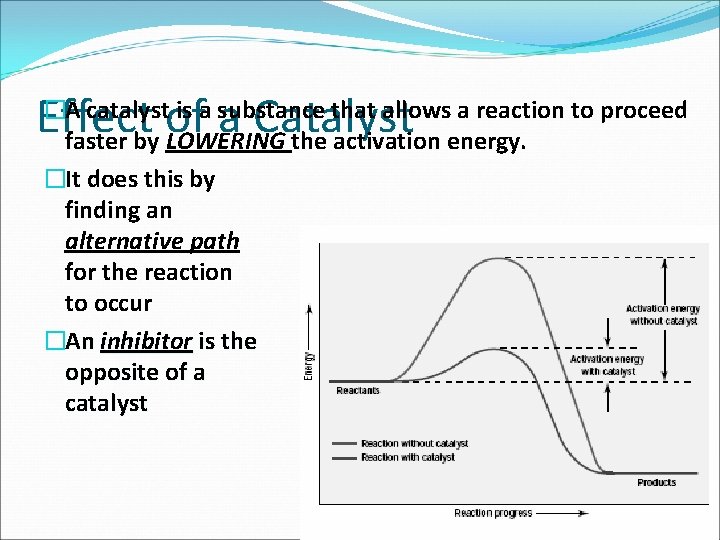
Effect of a Catalyst �A catalyst is a substance that allows a reaction to proceed faster by LOWERING the activation energy. �It does this by finding an alternative path for the reaction to occur �An inhibitor is the opposite of a catalyst
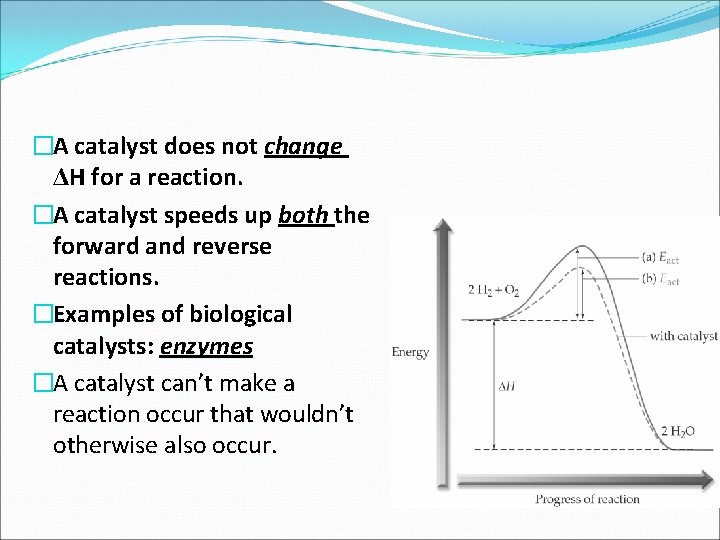
�A catalyst does not change ΔH for a reaction. �A catalyst speeds up both the forward and reverse reactions. �Examples of biological catalysts: enzymes �A catalyst can’t make a reaction occur that wouldn’t otherwise also occur.
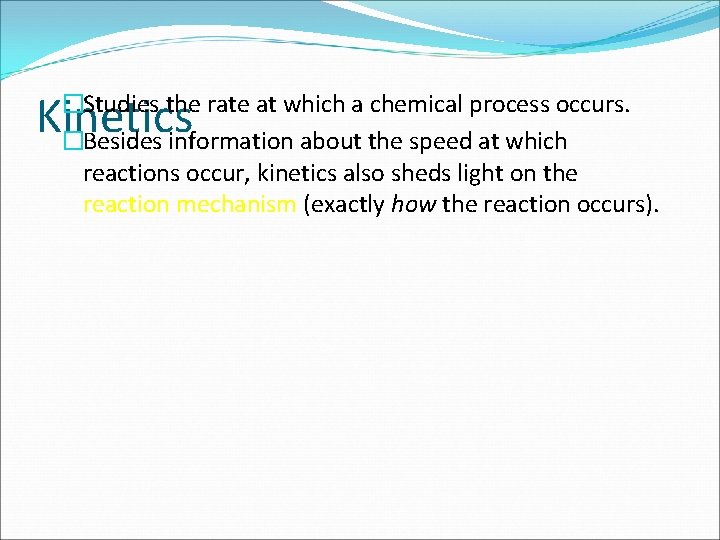
Kinetics �Studies the rate at which a chemical process occurs. �Besides information about the speed at which reactions occur, kinetics also sheds light on the reaction mechanism (exactly how the reaction occurs).
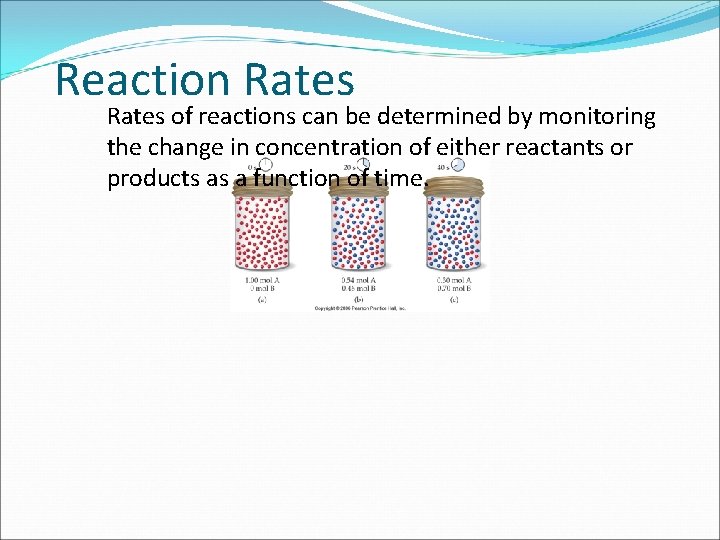
Reaction Rates of reactions can be determined by monitoring the change in concentration of either reactants or products as a function of time.
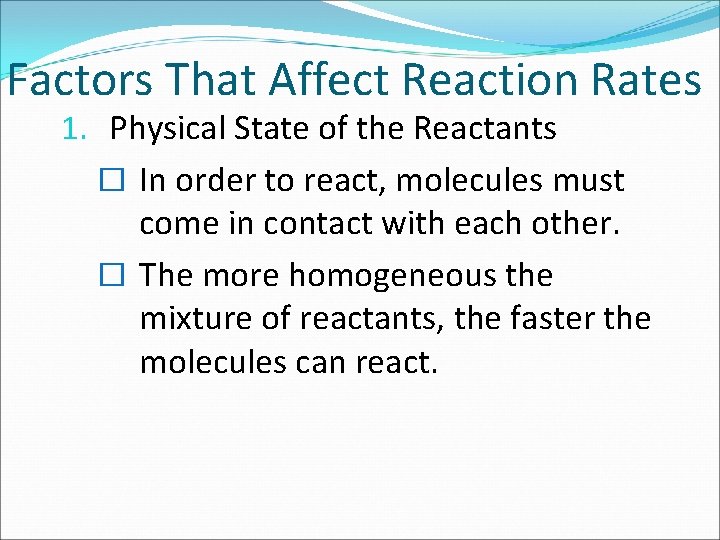
Factors That Affect Reaction Rates 1. Physical State of the Reactants � In order to react, molecules must come in contact with each other. � The more homogeneous the mixture of reactants, the faster the molecules can react.
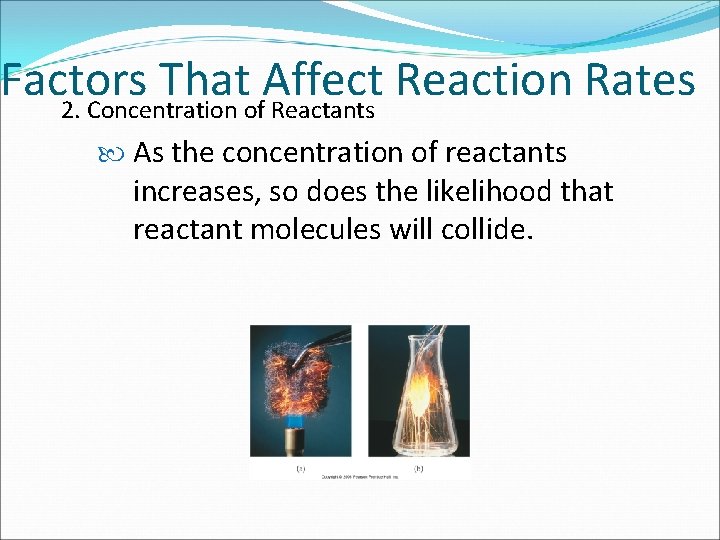
Factors That Affect Reaction Rates 2. Concentration of Reactants As the concentration of reactants increases, so does the likelihood that reactant molecules will collide.
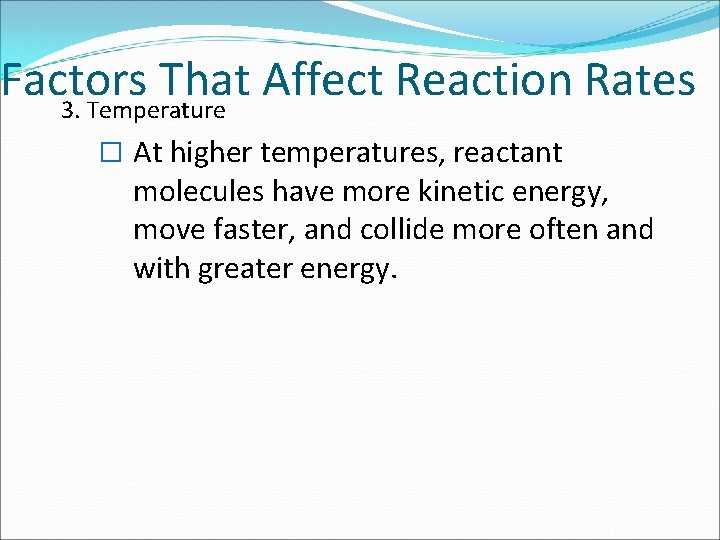
Factors That Affect Reaction Rates 3. Temperature � At higher temperatures, reactant molecules have more kinetic energy, move faster, and collide more often and with greater energy.
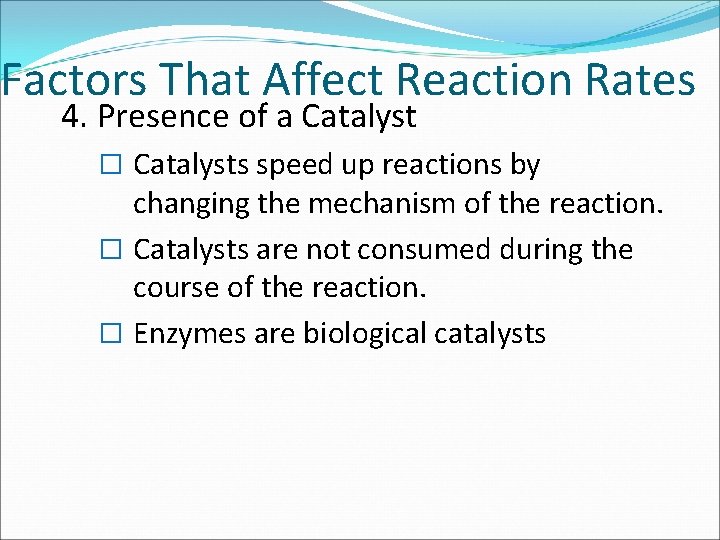
Factors That Affect Reaction Rates 4. Presence of a Catalyst � Catalysts speed up reactions by changing the mechanism of the reaction. � Catalysts are not consumed during the course of the reaction. � Enzymes are biological catalysts
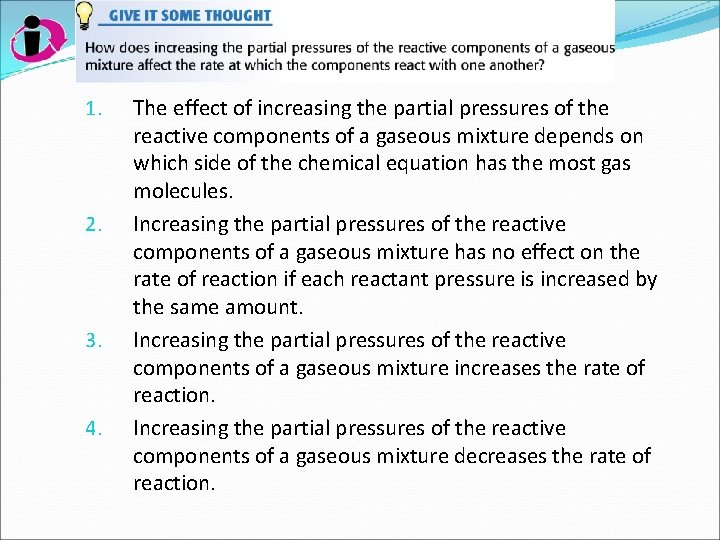
1. 2. 3. 4. The effect of increasing the partial pressures of the reactive components of a gaseous mixture depends on which side of the chemical equation has the most gas molecules. Increasing the partial pressures of the reactive components of a gaseous mixture has no effect on the rate of reaction if each reactant pressure is increased by the same amount. Increasing the partial pressures of the reactive components of a gaseous mixture increases the rate of reaction. Increasing the partial pressures of the reactive components of a gaseous mixture decreases the rate of reaction.
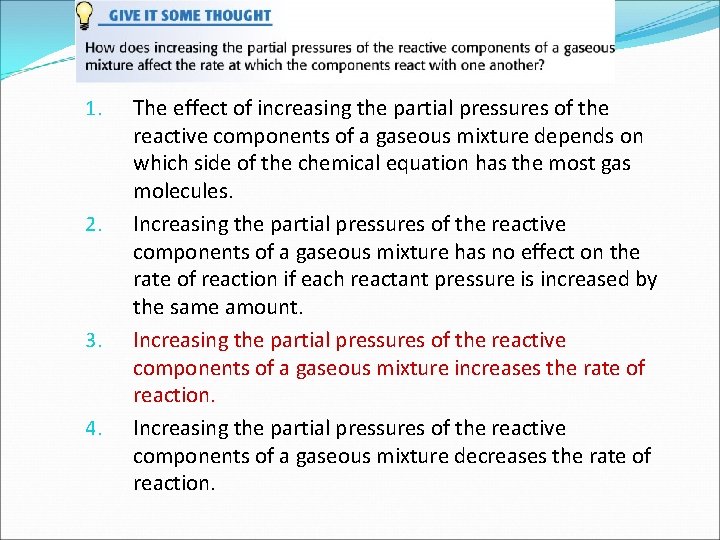
1. 2. 3. 4. The effect of increasing the partial pressures of the reactive components of a gaseous mixture depends on which side of the chemical equation has the most gas molecules. Increasing the partial pressures of the reactive components of a gaseous mixture has no effect on the rate of reaction if each reactant pressure is increased by the same amount. Increasing the partial pressures of the reactive components of a gaseous mixture increases the rate of reaction. Increasing the partial pressures of the reactive components of a gaseous mixture decreases the rate of reaction.
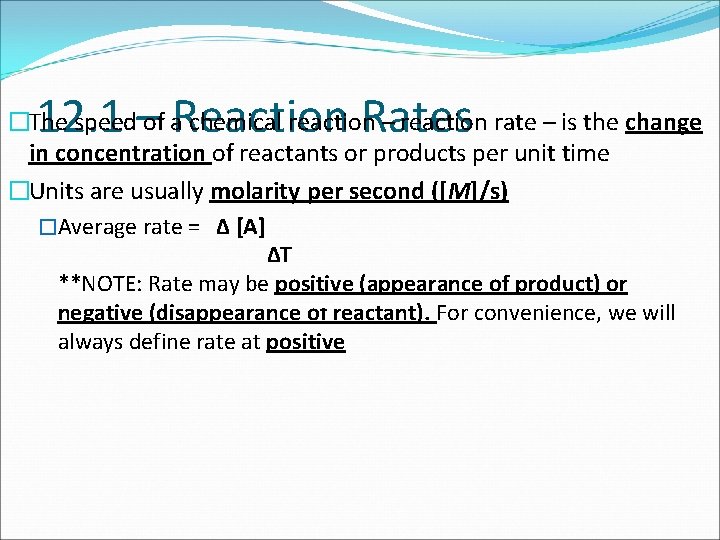
12. 1 – Reaction Rates �The speed of a chemical reaction – reaction rate – is the change in concentration of reactants or products per unit time �Units are usually molarity per second ([M]/s) �Average rate = Δ [A] ΔT **NOTE: Rate may be positive (appearance of product) or negative (disappearance of reactant). For convenience, we will always define rate at positive
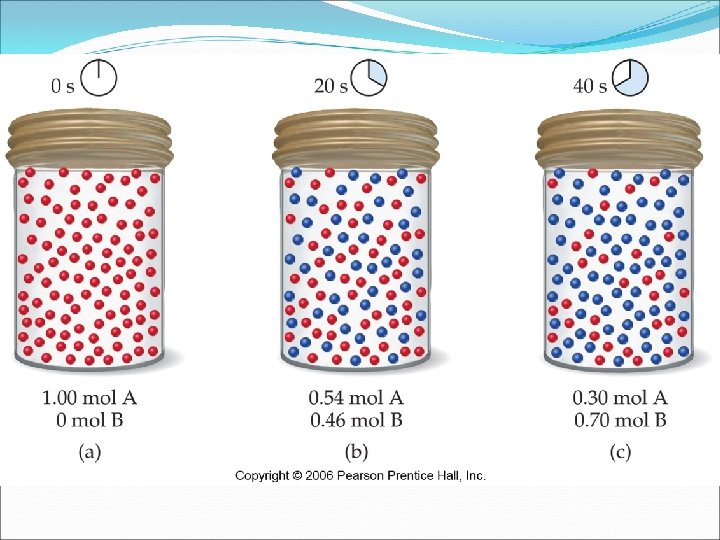
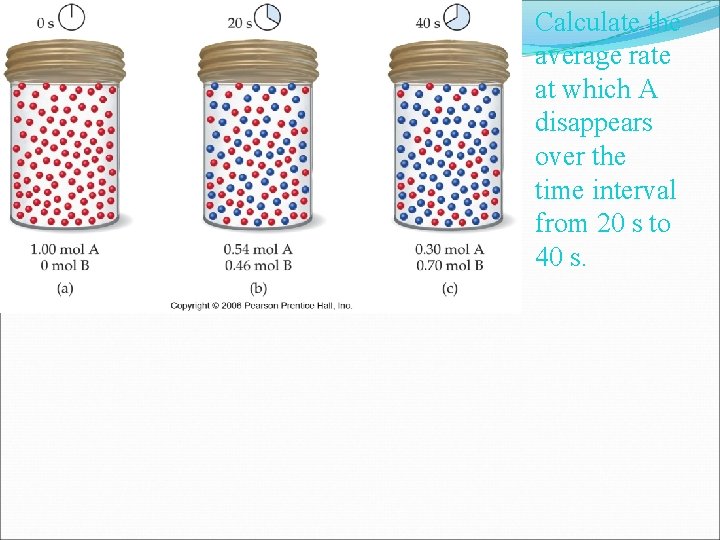
Calculate the average rate at which A disappears over the time interval from 20 s to 40 s.
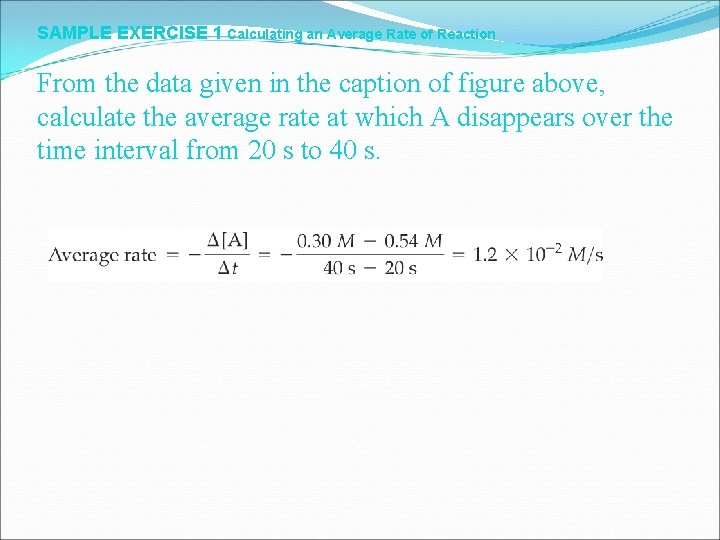
SAMPLE EXERCISE 1 Calculating an Average Rate of Reaction From the data given in the caption of figure above, calculate the average rate at which A disappears over the time interval from 20 s to 40 s.
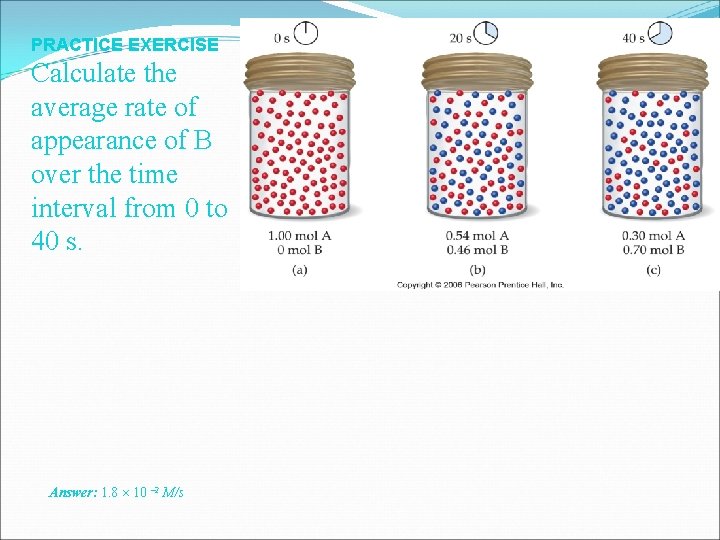
PRACTICE EXERCISE Calculate the average rate of appearance of B over the time interval from 0 to 40 s. Answer: 1. 8 10 – 2 M/s
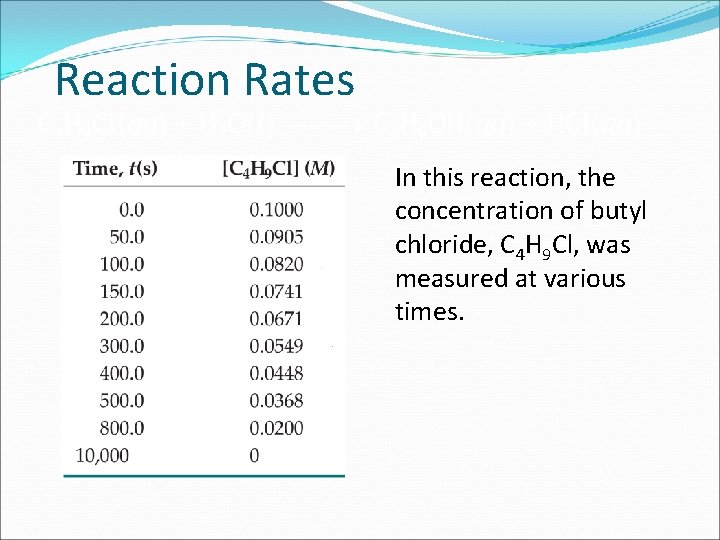
Reaction Rates C 4 H 9 Cl(aq) + H 2 O(l) C 4 H 9 OH(aq) + HCl(aq) In this reaction, the concentration of butyl chloride, C 4 H 9 Cl, was measured at various times.
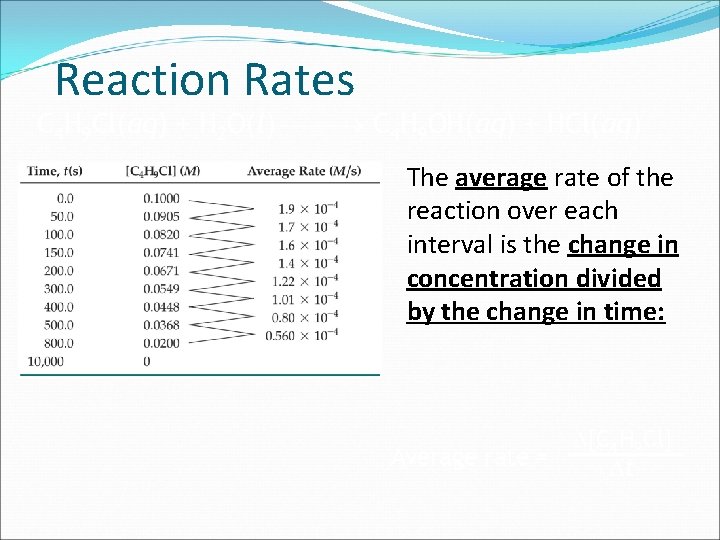
Reaction Rates C 4 H 9 Cl(aq) + H 2 O(l) C 4 H 9 OH(aq) + HCl(aq) The average rate of the reaction over each interval is the change in concentration divided by the change in time: [C 4 H 9 Cl] Average rate = t
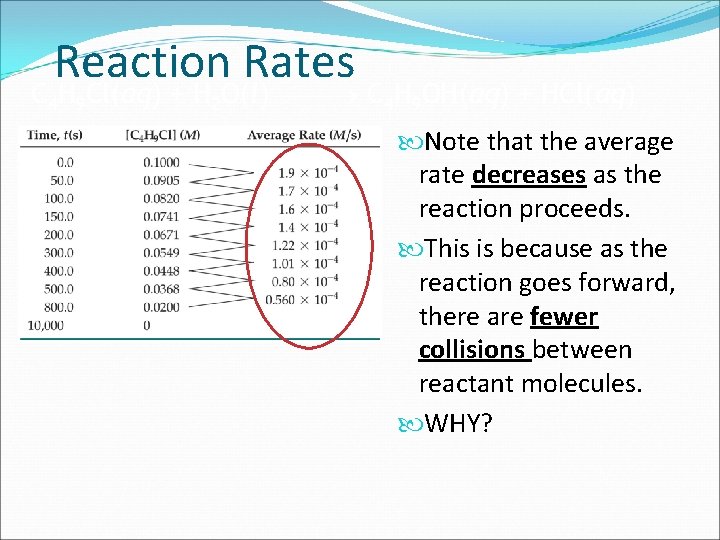
Reaction Rates C 4 H 9 Cl(aq) + H 2 O(l) C 4 H 9 OH(aq) + HCl(aq) Note that the average rate decreases as the reaction proceeds. This is because as the reaction goes forward, there are fewer collisions between reactant molecules. WHY?
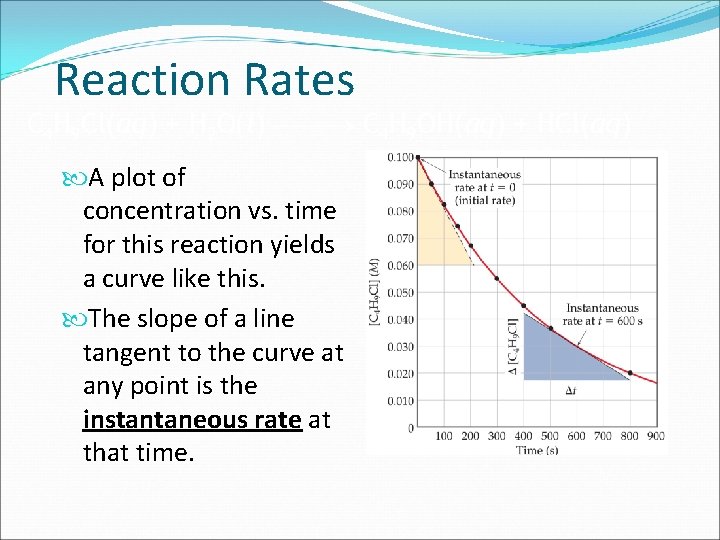
Reaction Rates C 4 H 9 Cl(aq) + H 2 O(l) C 4 H 9 OH(aq) + HCl(aq) A plot of concentration vs. time for this reaction yields a curve like this. The slope of a line tangent to the curve at any point is the instantaneous rate at that time.
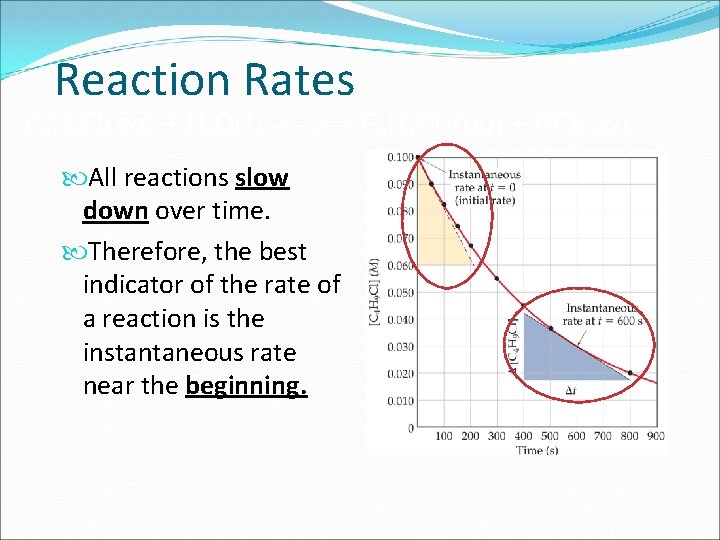
Reaction Rates C 4 H 9 Cl(aq) + H 2 O(l) C 4 H 9 OH(aq) + HCl(aq) All reactions slow down over time. Therefore, the best indicator of the rate of a reaction is the instantaneous rate near the beginning.
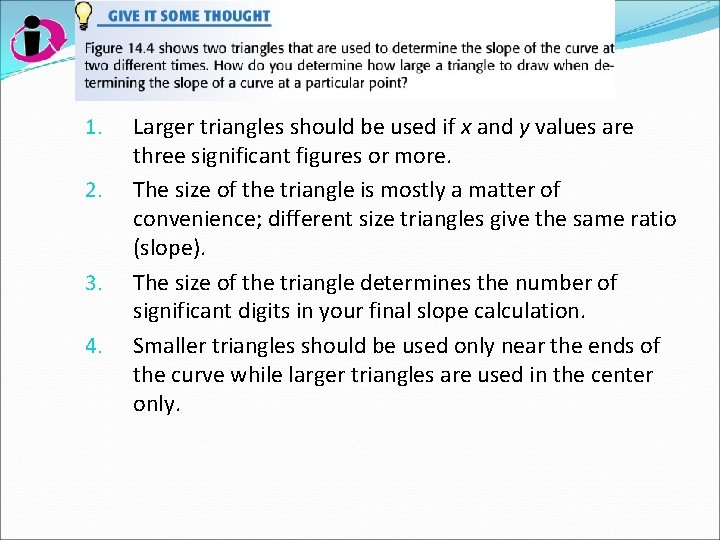
1. 2. 3. 4. Larger triangles should be used if x and y values are three significant figures or more. The size of the triangle is mostly a matter of convenience; different size triangles give the same ratio (slope). The size of the triangle determines the number of significant digits in your final slope calculation. Smaller triangles should be used only near the ends of the curve while larger triangles are used in the center only.
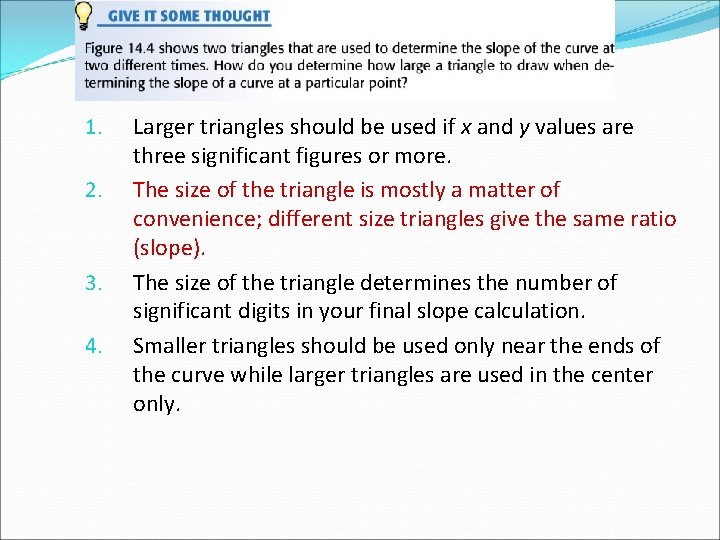
1. 2. 3. 4. Larger triangles should be used if x and y values are three significant figures or more. The size of the triangle is mostly a matter of convenience; different size triangles give the same ratio (slope). The size of the triangle determines the number of significant digits in your final slope calculation. Smaller triangles should be used only near the ends of the curve while larger triangles are used in the center only.
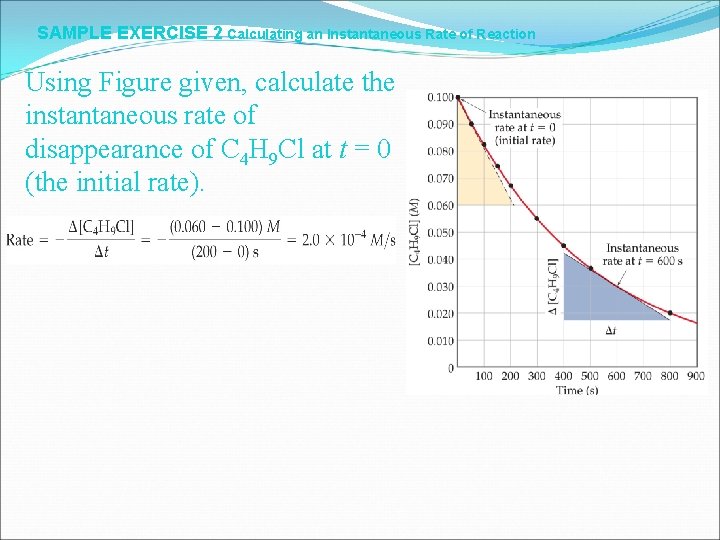
SAMPLE EXERCISE 2 Calculating an Instantaneous Rate of Reaction Using Figure given, calculate the instantaneous rate of disappearance of C 4 H 9 Cl at t = 0 (the initial rate).
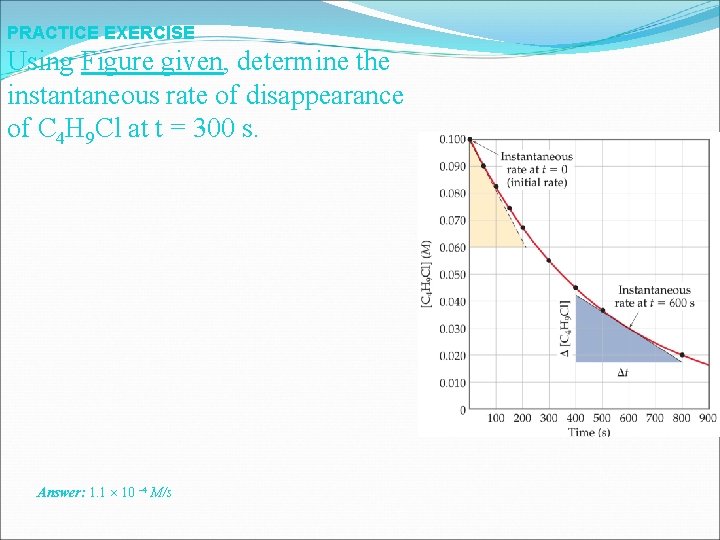
PRACTICE EXERCISE Using Figure given, determine the instantaneous rate of disappearance of C 4 H 9 Cl at t = 300 s. Answer: 1. 1 10 – 4 M/s
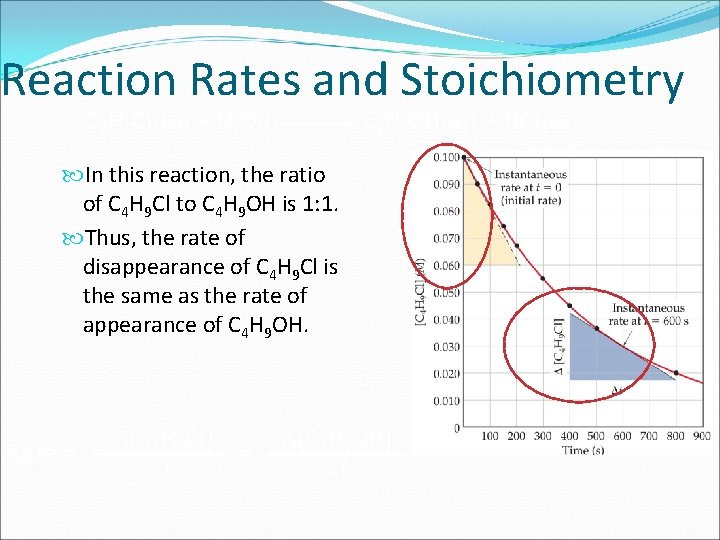
Reaction Rates and Stoichiometry C 4 H 9 Cl(aq) + H 2 O(l) C 4 H 9 OH(aq) + HCl(aq) In this reaction, the ratio of C 4 H 9 Cl to C 4 H 9 OH is 1: 1. Thus, the rate of disappearance of C 4 H 9 Cl is the same as the rate of appearance of C 4 H 9 OH. Rate = - [C 4 H 9 Cl] = t [C 4 H 9 OH] t
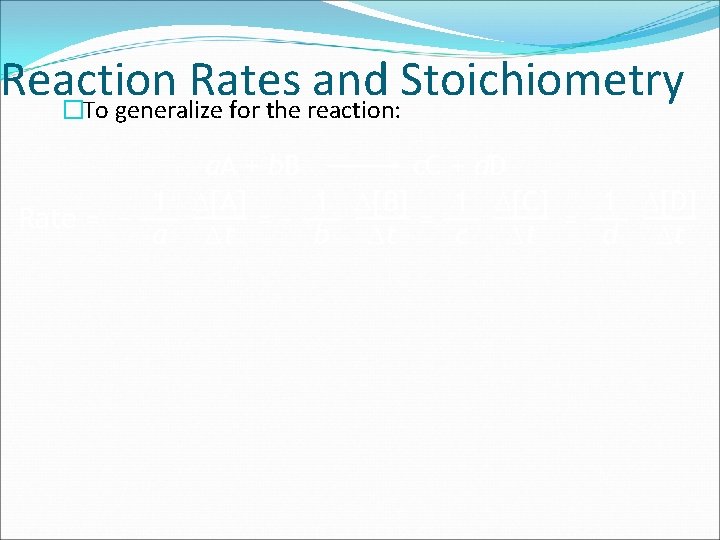
Reaction Rates and Stoichiometry �To generalize for the reaction: a. A + b. B c. C + d. D 1 [B] 1 [D] 1 [A] 1 [C] = = Rate = − =− b t d t a t c t
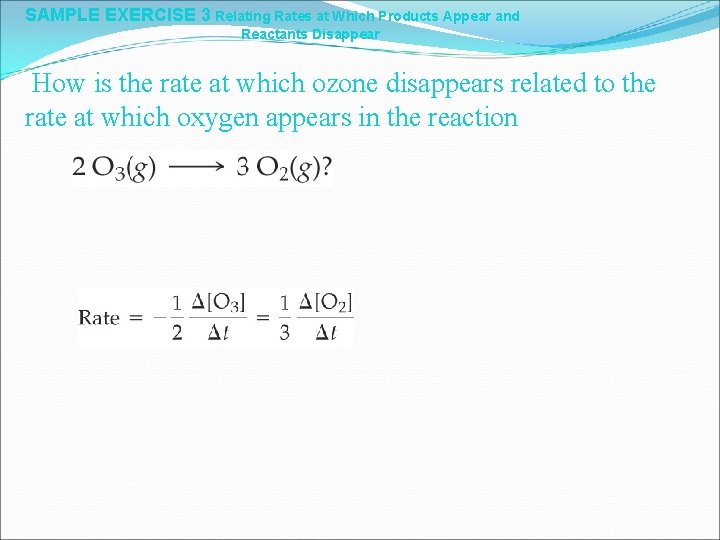
SAMPLE EXERCISE 3 Relating Rates at Which Products Appear and Reactants Disappear How is the rate at which ozone disappears related to the rate at which oxygen appears in the reaction
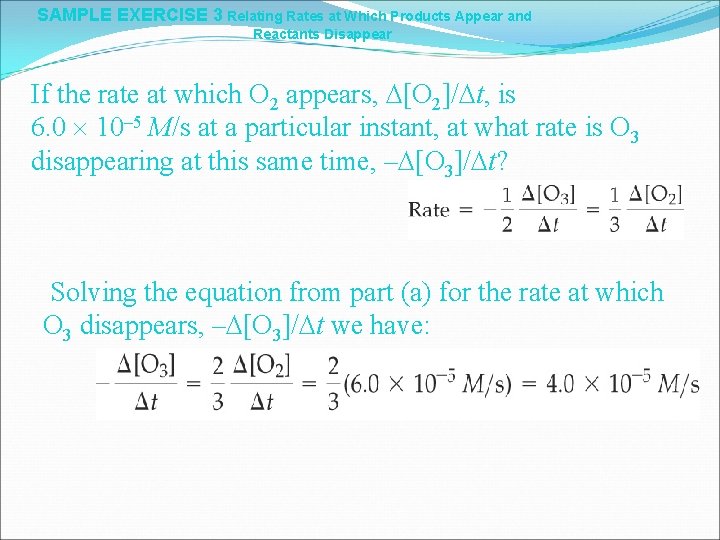
SAMPLE EXERCISE 3 Relating Rates at Which Products Appear and Reactants Disappear If the rate at which O 2 appears, [O 2]/ t, is 6. 0 10– 5 M/s at a particular instant, at what rate is O 3 disappearing at this same time, – [O 3]/ t? Solving the equation from part (a) for the rate at which O 3 disappears, – [O 3]/ t we have:
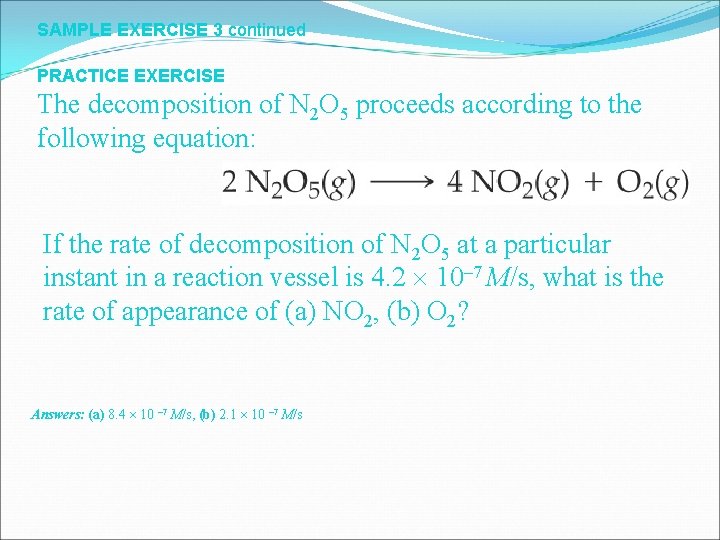
SAMPLE EXERCISE 3 continued PRACTICE EXERCISE The decomposition of N 2 O 5 proceeds according to the following equation: If the rate of decomposition of N 2 O 5 at a particular instant in a reaction vessel is 4. 2 10– 7 M/s, what is the rate of appearance of (a) NO 2, (b) O 2? Answers: (a) 8. 4 10 – 7 M/s, (b) 2. 1 10 – 7 M/s
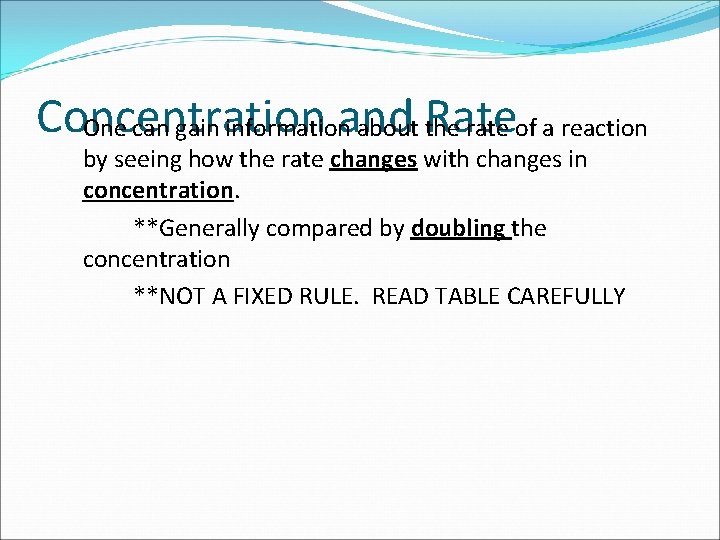
Concentration and Rate One can gain information about the rate of a reaction by seeing how the rate changes with changes in concentration. **Generally compared by doubling the concentration **NOT A FIXED RULE. READ TABLE CAREFULLY
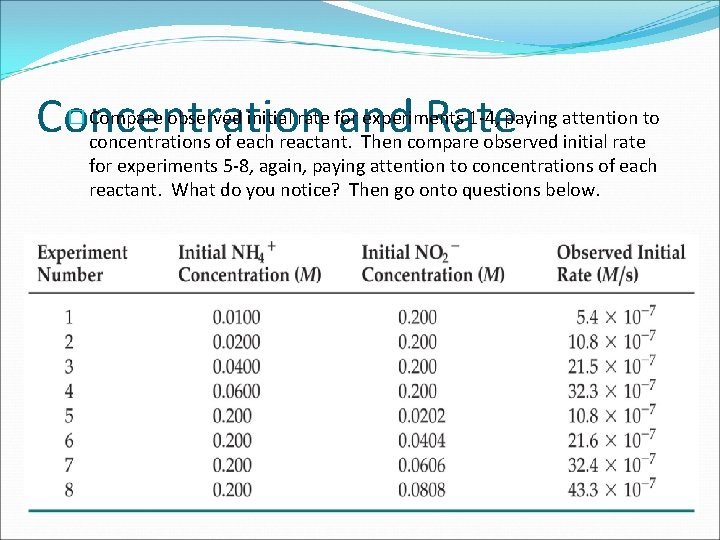
Concentration and Rate � Compare observed initial rate for experiments 1 -4, paying attention to concentrations of each reactant. Then compare observed initial rate for experiments 5 -8, again, paying attention to concentrations of each reactant. What do you notice? Then go onto questions below.
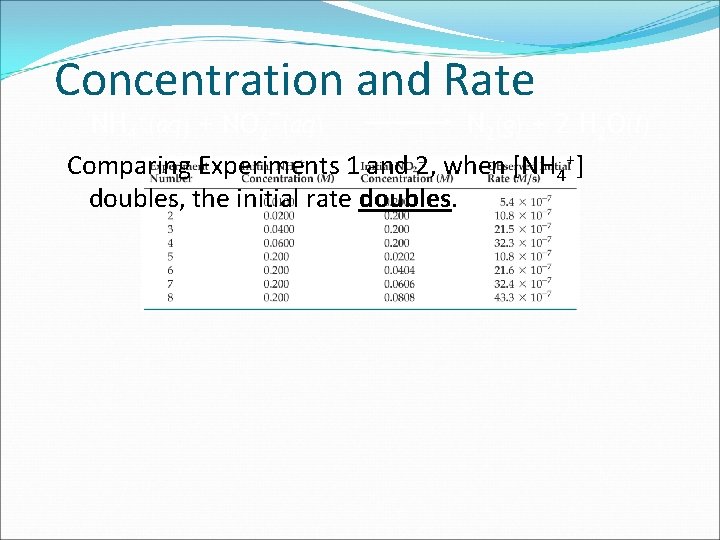
Concentration and Rate N 2(g) + 2 H 2 O(l) NH 4+(aq) + NO 2−(aq) Comparing Experiments 1 and 2, when [NH 4+] doubles, the initial rate doubles.
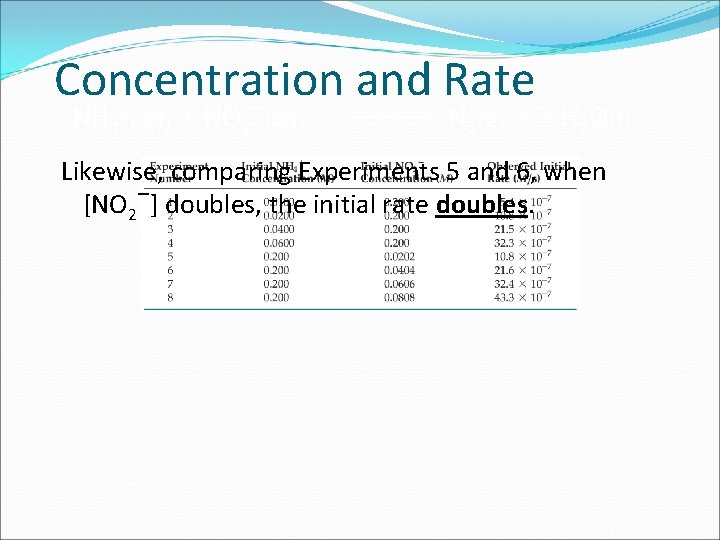
Concentration and Rate − NH 4+(aq) + NO 2 (aq) N 2(g) + 2 H 2 O(l) Likewise, comparing Experiments 5 and 6, when [NO 2−] doubles, the initial rate doubles.
![This means Rate NH 4 Translation rate is DIRECTLY proportionate to concentration of This means Rate [NH 4+] Translation: rate is DIRECTLY proportionate to concentration of](https://slidetodoc.com/presentation_image_h/1c9abc1fe8d10b8ee4aadf33e9ca8558/image-62.jpg)
This means Rate [NH 4+] Translation: rate is DIRECTLY proportionate to concentration of NH+ Rate [NO 2−] Translation: rate is DIRECTLY proportionate to concentration of NO 2 - Rate [NH+] [NO 2−] Rate = k [NH 4+] [NO 2−] Rate is directly proportionate to the product of the concentrations of each reactant Rate equation shows relationship between rate constant and each reactant This equation is called the rate law, and k is known as the rate constant.
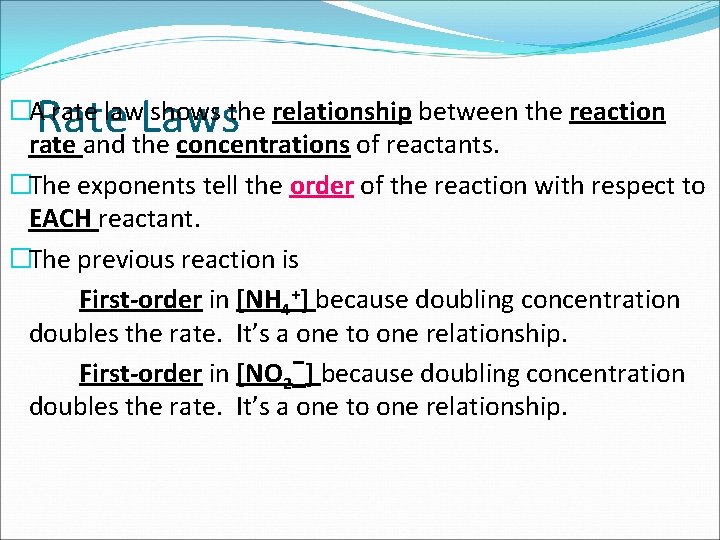
Rate Laws �A rate law shows the relationship between the reaction rate and the concentrations of reactants. �The exponents tell the order of the reaction with respect to EACH reactant. �The previous reaction is First-order in [NH 4+] because doubling concentration doubles the rate. It’s a one to one relationship. First-order in [NO 2−] because doubling concentration doubles the rate. It’s a one to one relationship.
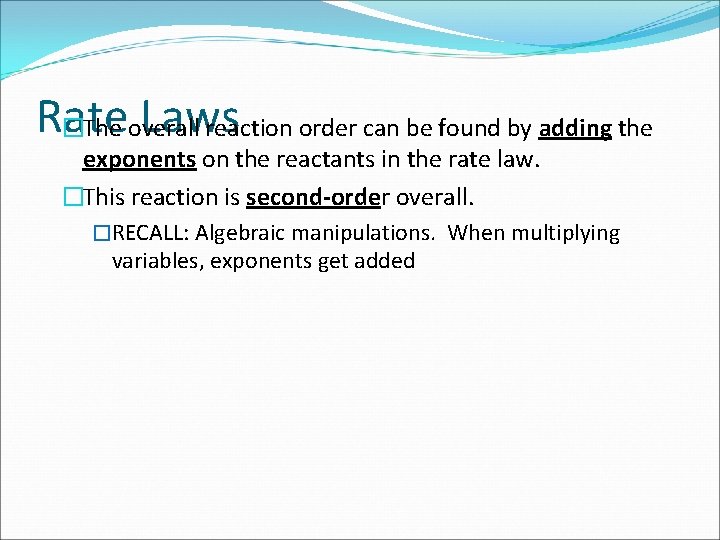
Rate Laws �The overall reaction order can be found by adding the exponents on the reactants in the rate law. �This reaction is second-order overall. �RECALL: Algebraic manipulations. When multiplying variables, exponents get added
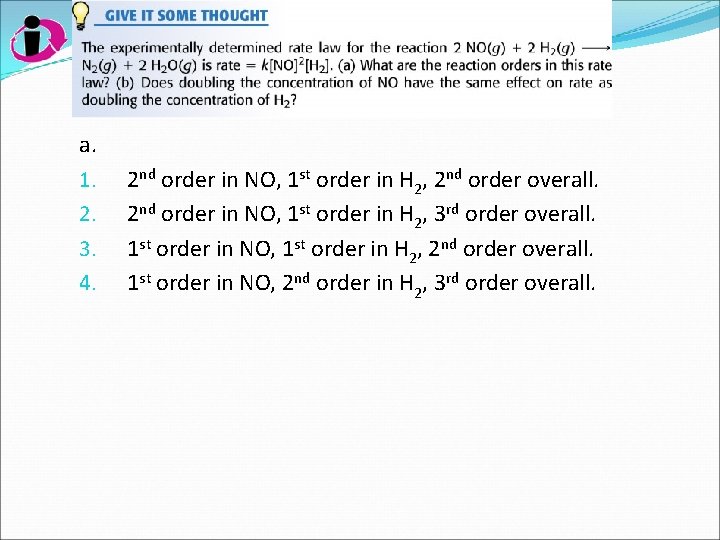
a. 1. 2. 3. 4. 2 nd order in NO, 1 st order in H 2, 2 nd order overall. 2 nd order in NO, 1 st order in H 2, 3 rd order overall. 1 st order in NO, 1 st order in H 2, 2 nd order overall. 1 st order in NO, 2 nd order in H 2, 3 rd order overall.
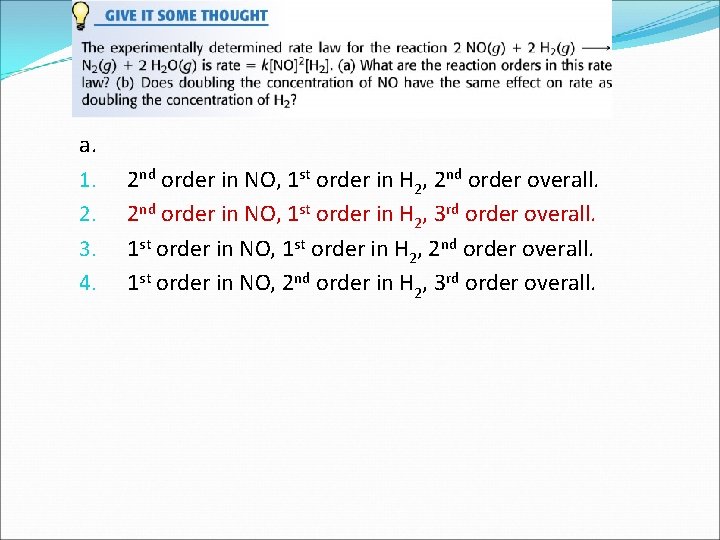
a. 1. 2. 3. 4. 2 nd order in NO, 1 st order in H 2, 2 nd order overall. 2 nd order in NO, 1 st order in H 2, 3 rd order overall. 1 st order in NO, 1 st order in H 2, 2 nd order overall. 1 st order in NO, 2 nd order in H 2, 3 rd order overall.
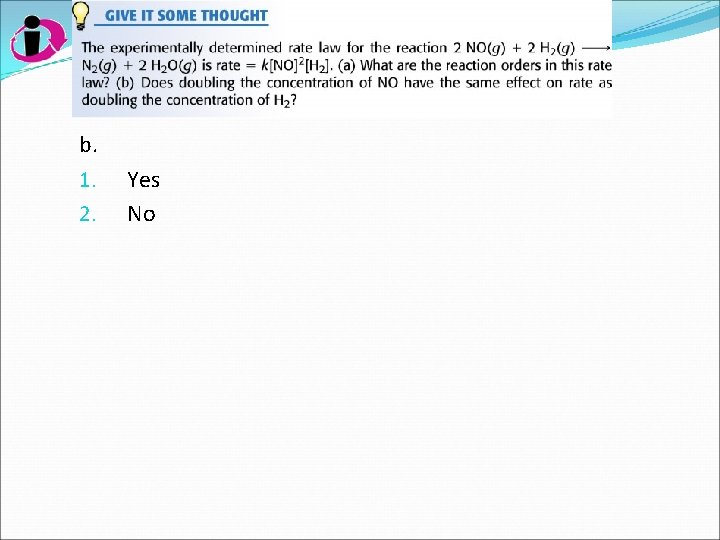
b. 1. 2. Yes No
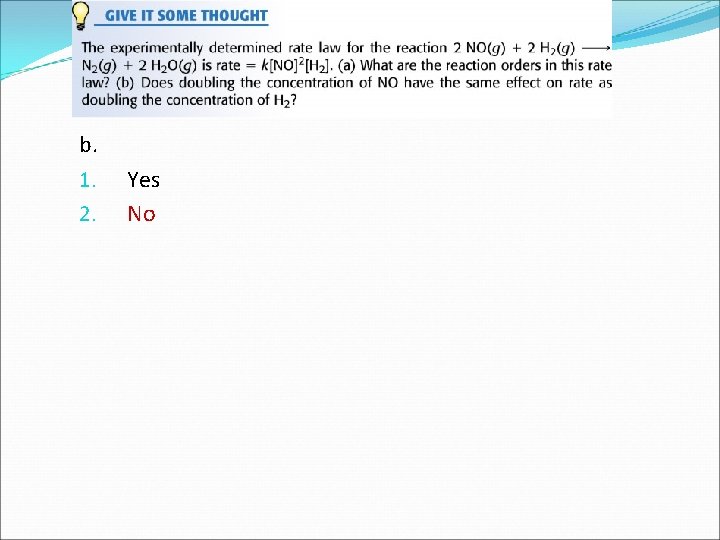
b. 1. 2. Yes No
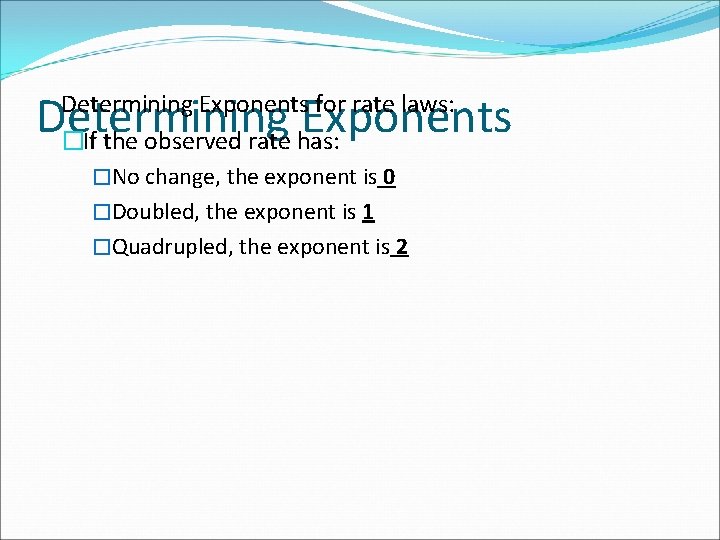
Determining Exponents for rate laws: �If the observed rate has: �No change, the exponent is 0 �Doubled, the exponent is 1 �Quadrupled, the exponent is 2
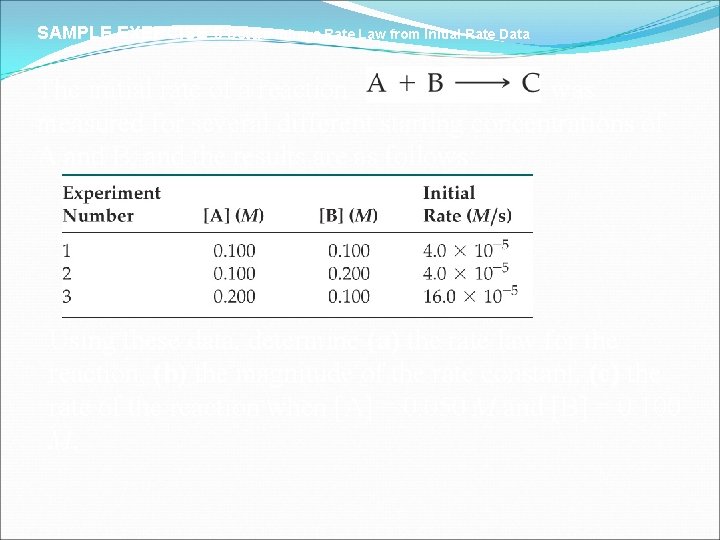
SAMPLE EXERCISE 6 Determining a Rate Law from Initial Rate Data The initial rate of a reaction was measured for several different starting concentrations of A and B, and the results are as follows: Using these data, determine (a) the rate law for the reaction, (b) the magnitude of the rate constant, (c) the rate of the reaction when [A] = 0. 050 M and [B] = 0. 100 M.
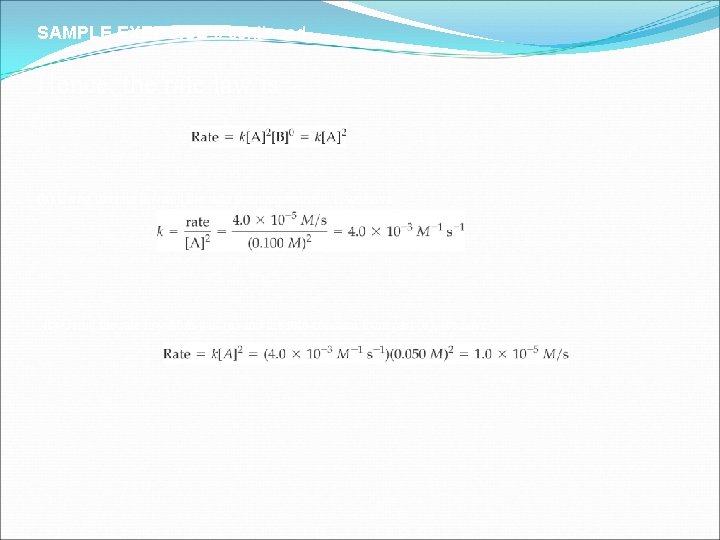
SAMPLE EXERCISE 6 continued Hence, the rate law is (b) Using the rate law and the data from experiment 1, we have (c) Using the rate law from part (a) and the rate constant from part (b), we have
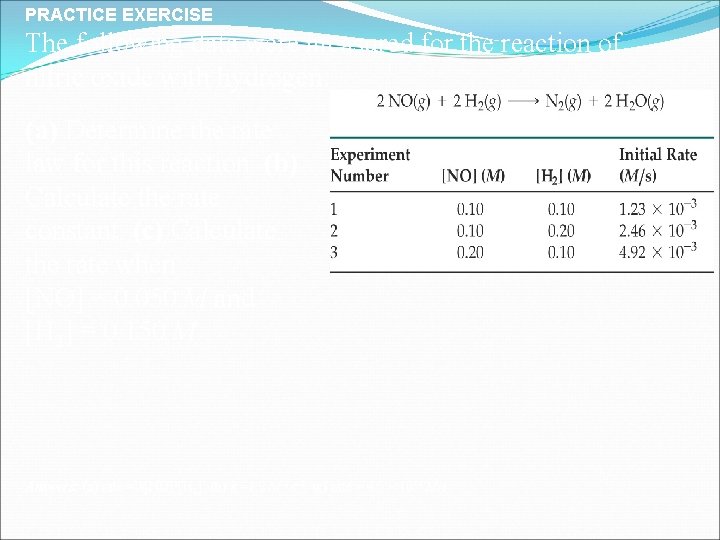
PRACTICE EXERCISE The following data were measured for the reaction of nitric oxide with hydrogen: (a) Determine the rate law for this reaction. (b) Calculate the rate constant. (c) Calculate the rate when [NO] = 0. 050 M and [H 2] = 0. 150 M. Answers: (a) rate = k[NO]2[H 2]; (b) k =1. 2 M– 2 s– 1; (c) rate = 4. 5 10– 4 M/s
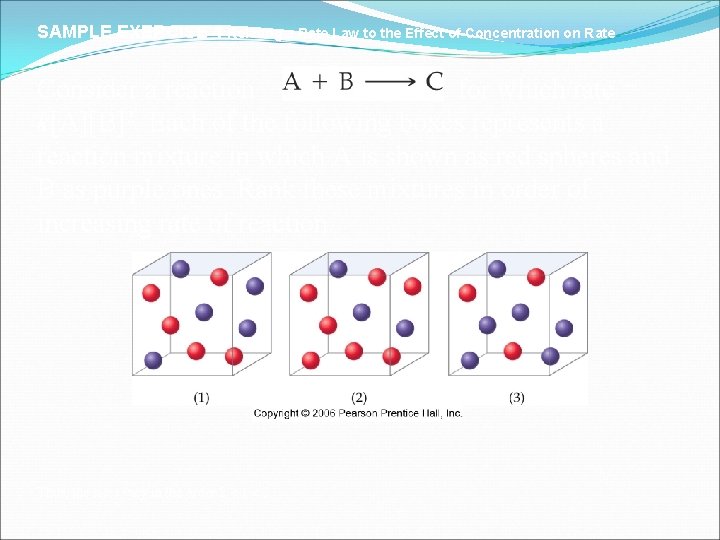
SAMPLE EXERCISE 4 Relating a Rate Law to the Effect of Concentration on Rate Consider a reaction for which rate = k[A][B]2. Each of the following boxes represents a reaction mixture in which A is shown as red spheres and B as purple ones. Rank these mixtures in order of increasing rate of reaction. Thus, the rates vary in the order 2 < 1 < 3.
![PRACTICE EXERCISE Assuming that rate kAB rank the mixtures represented in this Sample PRACTICE EXERCISE Assuming that rate = k[A][B], rank the mixtures represented in this Sample](https://slidetodoc.com/presentation_image_h/1c9abc1fe8d10b8ee4aadf33e9ca8558/image-74.jpg)
PRACTICE EXERCISE Assuming that rate = k[A][B], rank the mixtures represented in this Sample Exercise in order of increasing rate. Answer: 2 = 3 < 1
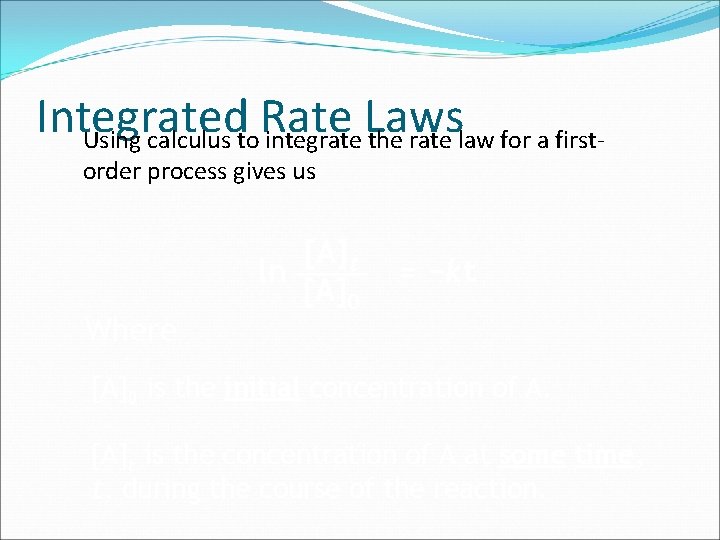
Integrated Rate Laws Using calculus to integrate the rate law for a firstorder process gives us Where [A]t ln [A]0 = −kt [A]0 is the initial concentration of A. [A]t is the concentration of A at some time, t, during the course of the reaction.
![Manipulating this equation Integrated Rateproduces Laws At ln kt A0 ln At Manipulating this equation Integrated Rateproduces… Laws [A]t ln = −kt [A]0 ln [A]t −](https://slidetodoc.com/presentation_image_h/1c9abc1fe8d10b8ee4aadf33e9ca8558/image-76.jpg)
Manipulating this equation Integrated Rateproduces… Laws [A]t ln = −kt [A]0 ln [A]t − ln [A]0 = − kt ln [A]t = − kt + ln [A]0 …which is in the form y = mx + b
![FirstOrder Processes Therefore if a reaction is firstorder a plot of ln A vs First-Order Processes Therefore, if a reaction is first-order, a plot of ln [A] vs.](https://slidetodoc.com/presentation_image_h/1c9abc1fe8d10b8ee4aadf33e9ca8558/image-77.jpg)
First-Order Processes Therefore, if a reaction is first-order, a plot of ln [A] vs. t t will yield a straight line, and the slope of the line will be k. Graph of: ln [A]t = -kt + ln [A]0 ln[A]t – ln[A]0 = -kt
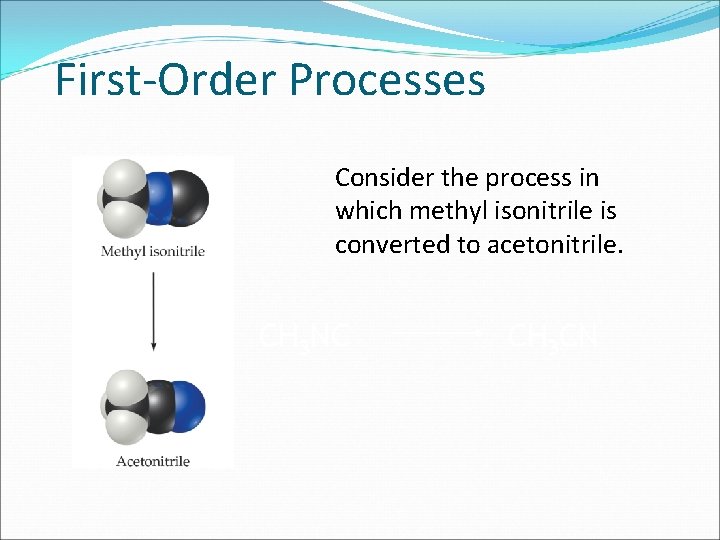
First-Order Processes Consider the process in which methyl isonitrile is converted to acetonitrile. CH 3 NC CH 3 CN
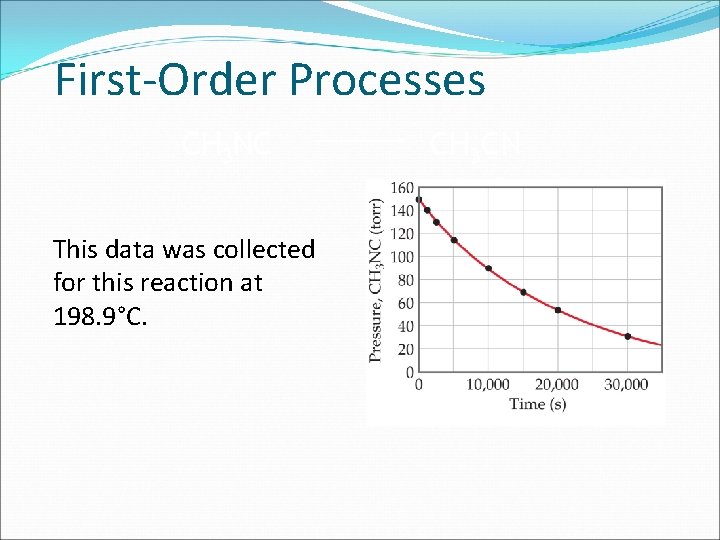
First-Order Processes CH 3 NC This data was collected for this reaction at 198. 9°C. CH 3 CN
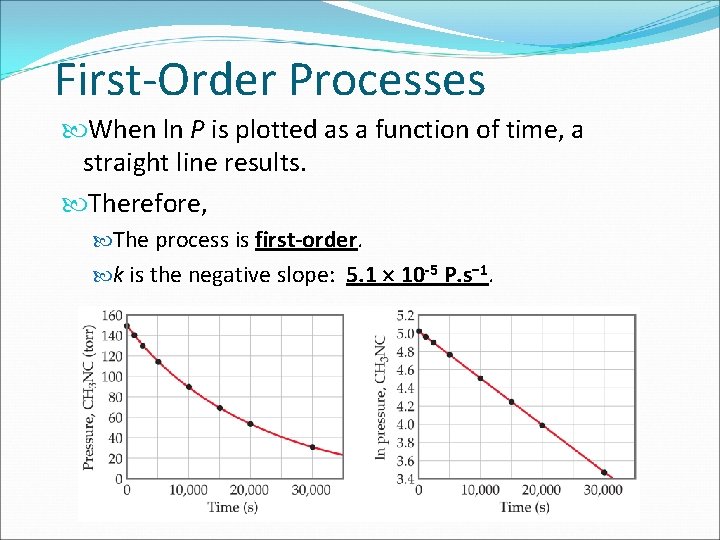
First-Order Processes When ln P is plotted as a function of time, a straight line results. Therefore, The process is first-order. k is the negative slope: 5. 1 10 -5 P. s− 1.
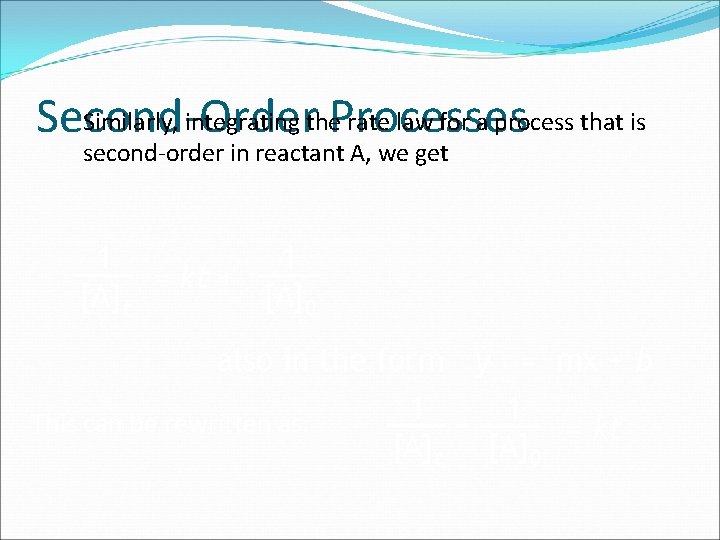
Similarly, integrating the. Processes rate law for a process that is Second-Order second-order in reactant A, we get 1 1 = kt + [A]t [A]0 also in the form y = mx + b This can be rewritten as: 1 [A]t 1 = kt [A]0
![SecondOrder Processes 1 1 At kt A0 So if a process is Second-Order Processes 1 1 [A]t = kt + [A]0 So if a process is](https://slidetodoc.com/presentation_image_h/1c9abc1fe8d10b8ee4aadf33e9ca8558/image-82.jpg)
Second-Order Processes 1 1 [A]t = kt + [A]0 So if a process is second-order in A, a plot of 1/[A]t vs. t will yield a straight line, and the slope of that line is k.
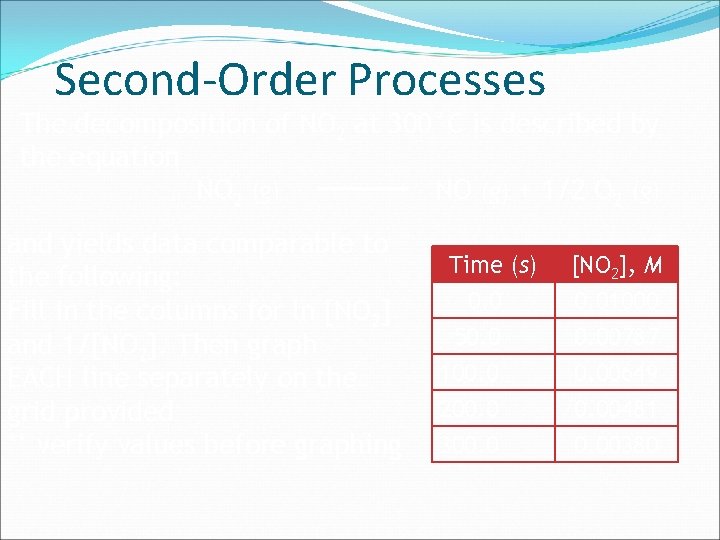
Second-Order Processes The decomposition of NO 2 at 300°C is described by the equation NO 2 (g) NO (g) + 1/2 O 2 (g) and yields data comparable to the following: Fill in the columns for ln [NO 2] and 1/[NO 2]. Then graph EACH line separately on the grid provided ** verify values before graphing Time (s) 0. 0 50. 0 100. 0 [NO 2], M 0. 01000 0. 00787 0. 00649 200. 0 300. 00481 0. 00380
![Time s NO 2 M ln NO 2 1NO 2 0 01000 4 Time (s) [NO 2], M ln [NO 2] 1/[NO 2] 0. 01000 − 4.](https://slidetodoc.com/presentation_image_h/1c9abc1fe8d10b8ee4aadf33e9ca8558/image-84.jpg)
Time (s) [NO 2], M ln [NO 2] 1/[NO 2] 0. 01000 − 4. 610 100 50. 00787 − 4. 845 127 100. 00649 − 5. 038 154 200. 00481 − 5. 337 208 300. 00380 − 5. 573 263
![SecondOrder Processes Graphing ln NO 2 vs t yields The plot is Second-Order Processes • Graphing ln [NO 2] vs. t yields: • The plot is](https://slidetodoc.com/presentation_image_h/1c9abc1fe8d10b8ee4aadf33e9ca8558/image-85.jpg)
Second-Order Processes • Graphing ln [NO 2] vs. t yields: • The plot is not a straight line, so the process is not first-order in [A]. Time (s) 0. 0 50. 0 100. 0 [NO 2], M 0. 01000 0. 00787 0. 00649 ln [NO 2] − 4. 610 − 4. 845 − 5. 038 200. 0 300. 00481 0. 00380 − 5. 337 − 5. 573
![SecondOrder Processes Graphing ln 1NO 2 vs t however gives this plot Time Second-Order Processes • Graphing ln 1/[NO 2] vs. t, however, gives this plot. Time](https://slidetodoc.com/presentation_image_h/1c9abc1fe8d10b8ee4aadf33e9ca8558/image-86.jpg)
Second-Order Processes • Graphing ln 1/[NO 2] vs. t, however, gives this plot. Time (s) 0. 0 50. 0 100. 0 [NO 2], M 0. 01000 0. 00787 0. 00649 1/[NO 2] 100 127 154 200. 00481 208 300. 00380 263 • Because this is a straight line, the process is secondorder in [A].
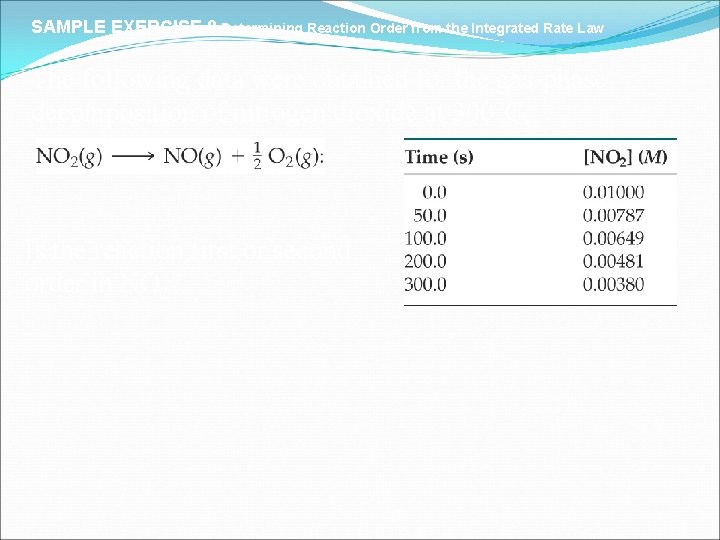
SAMPLE EXERCISE 8 Determining Reaction Order from the Integrated Rate Law The following data were obtained for the gas-phase decomposition of nitrogen dioxide at 300°C, Is the reaction first or second order in NO 2?
![SAMPLE EXERCISE 8 continued In order to graph lnNO 2 and 1NO 2 against SAMPLE EXERCISE 8 continued In order to graph ln[NO 2] and 1/[NO 2] against](https://slidetodoc.com/presentation_image_h/1c9abc1fe8d10b8ee4aadf33e9ca8558/image-88.jpg)
SAMPLE EXERCISE 8 continued In order to graph ln[NO 2] and 1/[NO 2] against time, we will first prepare the following table from the data given: Solve: Now draw two separate graphs for ln[NO 2] and 1/[NO 2]
![SAMPLE EXERCISE 8 continued As Figure below shows only the plot of 1NO 2 SAMPLE EXERCISE 8 continued As Figure below shows, only the plot of 1/[NO 2]](https://slidetodoc.com/presentation_image_h/1c9abc1fe8d10b8ee4aadf33e9ca8558/image-89.jpg)
SAMPLE EXERCISE 8 continued As Figure below shows, only the plot of 1/[NO 2] versus time is linear. Thus, the reaction obeys a second-order rate law: Rate = k[NO 2]2. From the slope of this straight-line graph, we determine that k = 0. 543 M– 1 s– 1 for the disappearance of NO 2. Figure Kinetic data for decomposition of NO 2. The reaction is NO 2(g) NO(g) + 1/2 O 2(g), and the data were collected at 300°C. (a) A plot of [NO 2] versus time is not linear, indicating that the reaction is not first order in NO 2. (b) A plot of 1/[NO 2] versus time is linear, indicating that the reaction is second order in NO 2. PRACTICE EXERCISE Consider again the decomposition of NO 2 discussed in the Sample Exercise. The reaction is second order in NO 2 with k = 0. 543 M– 1 s– 1. If the initial concentration of NO 2 in a closed vessel is 0. 0500 M, what is the remaining concentration after 0. 500 h? Answer: Using Equation given, we find [NO 2] = 1. 00 10– 3 M
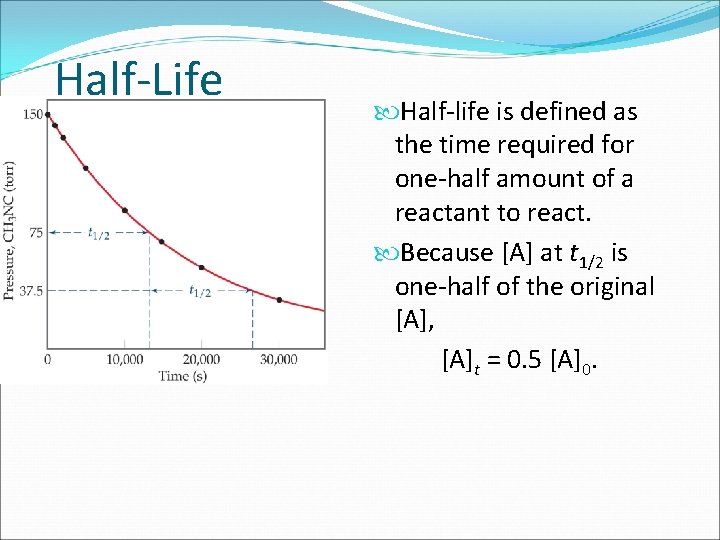
Half-Life Half-life is defined as the time required for one-half amount of a reactant to react. Because [A] at t 1/2 is one-half of the original [A], [A]t = 0. 5 [A]0.
![For a firstorder process this becomes HalfLife 0 5 A0 ln kt 12 For a first-order process, this becomes Half-Life 0. 5 [A]0 ln = −kt 1/2](https://slidetodoc.com/presentation_image_h/1c9abc1fe8d10b8ee4aadf33e9ca8558/image-91.jpg)
For a first-order process, this becomes Half-Life 0. 5 [A]0 ln = −kt 1/2 [A]0 NOTE: For a first-order process, the half-life does not depend on [A]0. ln 0. 5 = −kt 1/2 − 0. 693 = −kt 1/2 0. 693 = t 1/2 k
![For a secondorder process HalfLife 1 1 kt 12 0 5 A0 For a second-order process, Half-Life 1 1 = kt 1/2 + 0. 5 [A]0](https://slidetodoc.com/presentation_image_h/1c9abc1fe8d10b8ee4aadf33e9ca8558/image-92.jpg)
For a second-order process, Half-Life 1 1 = kt 1/2 + 0. 5 [A]0 2 1 = kt 1/2 + [A]0 2 − 1 = kt 1/2 [A]0 0 1 = t 1/2 k[A]0
![Graphs and Kinetic Data Plotting concentration of reactant REACTANT or other relevant value against Graphs and Kinetic Data �Plotting concentration of reactant [REACTANT] (or other relevant value) against](https://slidetodoc.com/presentation_image_h/1c9abc1fe8d10b8ee4aadf33e9ca8558/image-93.jpg)
Graphs and Kinetic Data �Plotting concentration of reactant [REACTANT] (or other relevant value) against time �One method of defining rate is to use the expression below, �Where gradient (slope or slope of tangent) of such a graph will equal the rate Rate = Change in concentration of reactant Time
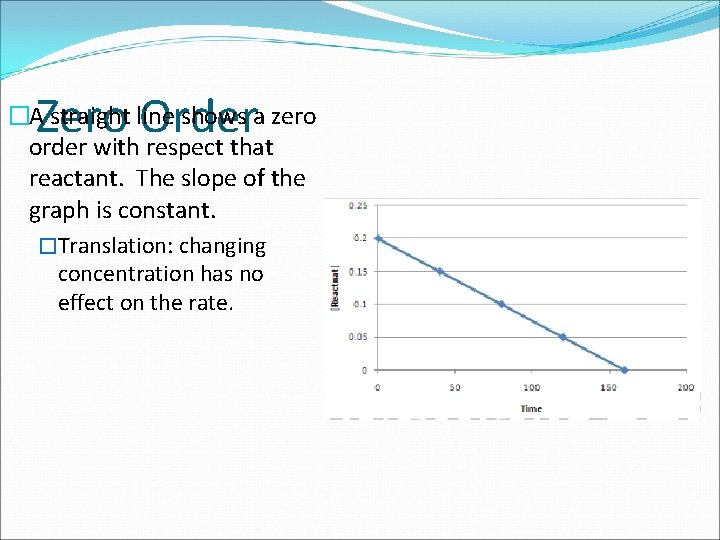
Zero Order �A straight line shows a zero order with respect that reactant. The slope of the graph is constant. �Translation: changing concentration has no effect on the rate.
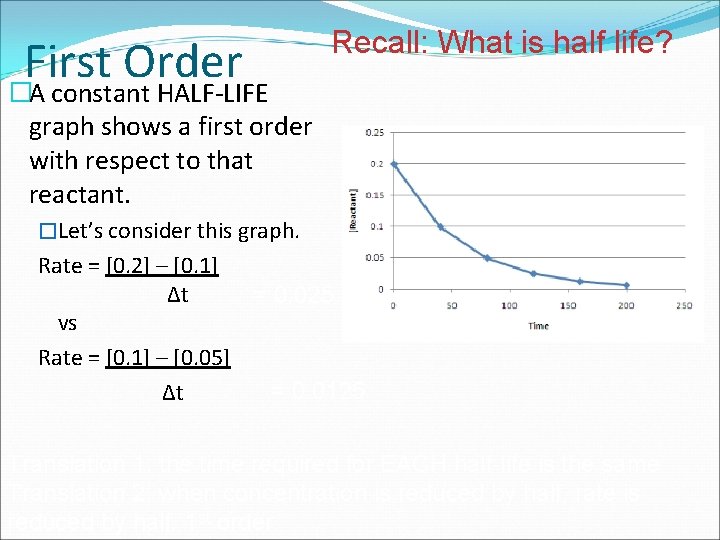
First Order Recall: What is half life? �A constant HALF-LIFE graph shows a first order with respect to that reactant. �Let’s consider this graph. Rate = [0. 2] – [0. 1] ∆t = 0. 025 vs Rate = [0. 1] – [0. 05] = 0. 0125 ∆t Translation 1: the time required for EACH half-life is the same Translation 2: when concentration is reduced by half, rate is reduced by half. 1 st order
![Second order Carefully note curve of Rate 0 2 0 1 t Second order �Carefully note: curve of Rate = [0. 2] – [0. 1] ∆t](https://slidetodoc.com/presentation_image_h/1c9abc1fe8d10b8ee4aadf33e9ca8558/image-96.jpg)
Second order �Carefully note: curve of Rate = [0. 2] – [0. 1] ∆t vs Rate = [0. 1] – [0. 05] ∆t 0. 2 [Reactant] graph is similar to order. BUT on closer inspection, note significant differences. Let’s consider rate 0. 25 1 st 0. 15 0. 1 0. 05 =0. 002 0 0 50 100 150 200 Time 250 300 350 400 = 0. 0005 Translation: Half life is NOT constant. Notice that it takes TWICE the amount of time to reduce concentration by HALF. When concentration is reduced by half, Rate is reduced to a quarter. That’s a factor of 4, making this a SECOND order
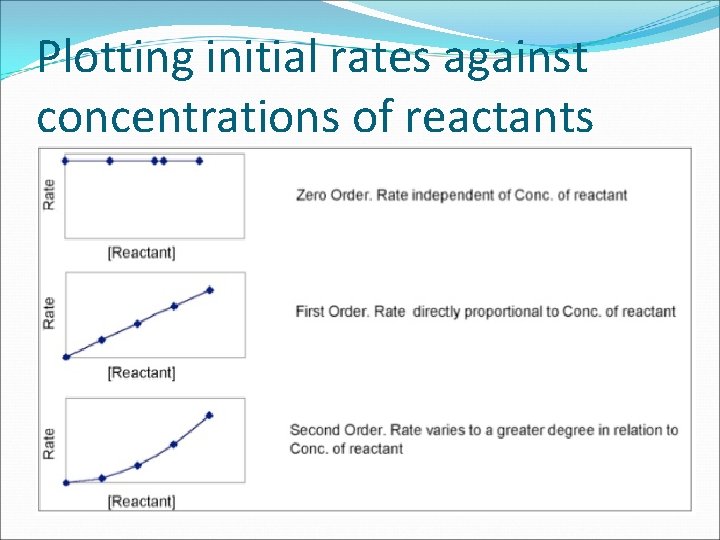
Plotting initial rates against concentrations of reactants
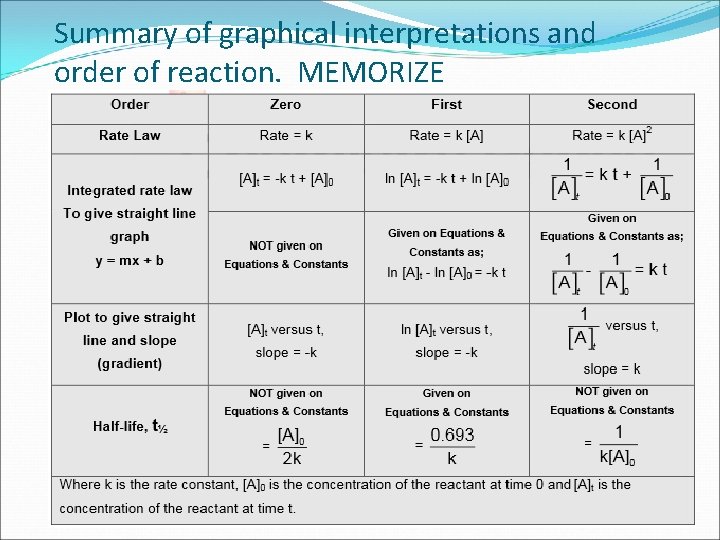
Summary of graphical interpretations and order of reaction. MEMORIZE
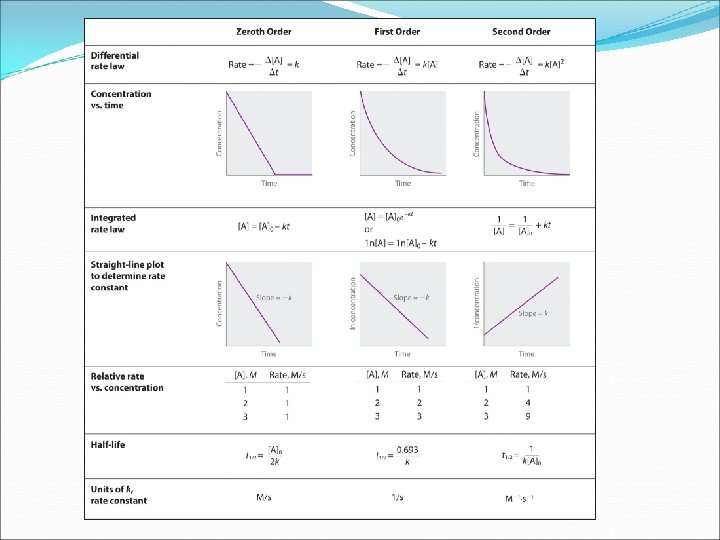
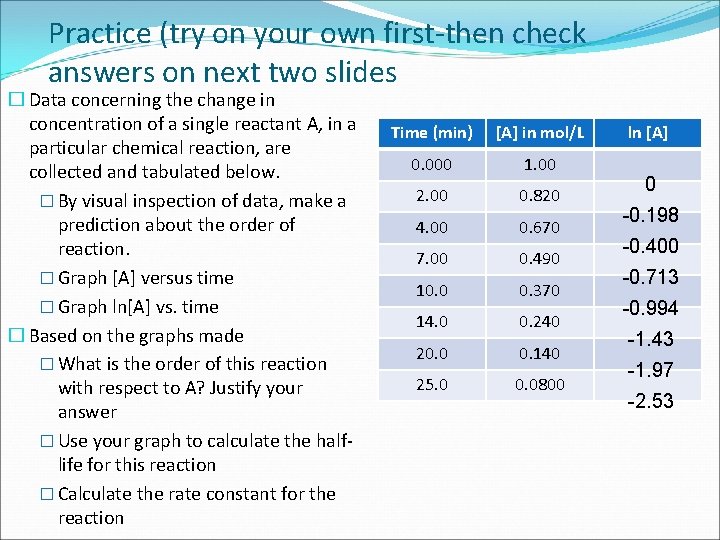
Practice (try on your own first-then check answers on next two slides � Data concerning the change in concentration of a single reactant A, in a particular chemical reaction, are collected and tabulated below. � By visual inspection of data, make a prediction about the order of reaction. � Graph [A] versus time � Graph ln[A] vs. time � Based on the graphs made � What is the order of this reaction with respect to A? Justify your answer � Use your graph to calculate the halflife for this reaction � Calculate the rate constant for the reaction Time (min) [A] in mol/L 0. 000 1. 00 2. 00 0. 820 4. 00 0. 670 7. 00 0. 490 10. 0 0. 370 14. 0 0. 240 20. 0 0. 140 25. 0 0. 0800 ln [A] 0 -0. 198 -0. 400 -0. 713 -0. 994 -1. 43 -1. 97 -2. 53
![1 2 0 time vs A 0 1 time lnA 20 10 vs 15 1. 2 0 time vs. [A] 0 1 time ln[A] 20 10 vs. 15](https://slidetodoc.com/presentation_image_h/1c9abc1fe8d10b8ee4aadf33e9ca8558/image-101.jpg)
1. 2 0 time vs. [A] 0 1 time ln[A] 20 10 vs. 15 25 30 -0. 5 0. 8 -1 0. 6 time vs. [A] 0. 4 -1. 5 ln [A] 5 time vs. ln[A] -2 0. 2 -2. 5 0 0 5 1. 10 20 25 30 -3 time 1 st order because a. b. 2. 3. 15 Time half life is constant (in first graph ∆ [] from 1 to 0. 490 takes 7 minutes, and from 0. 490 to 0. 240 takes 7 minutes. Graph of time vs. ln[A] is a straight line with negative slope, which corresponds to a 1 st order reaction. Half life is 7 minutes Rate constant (see next slide)
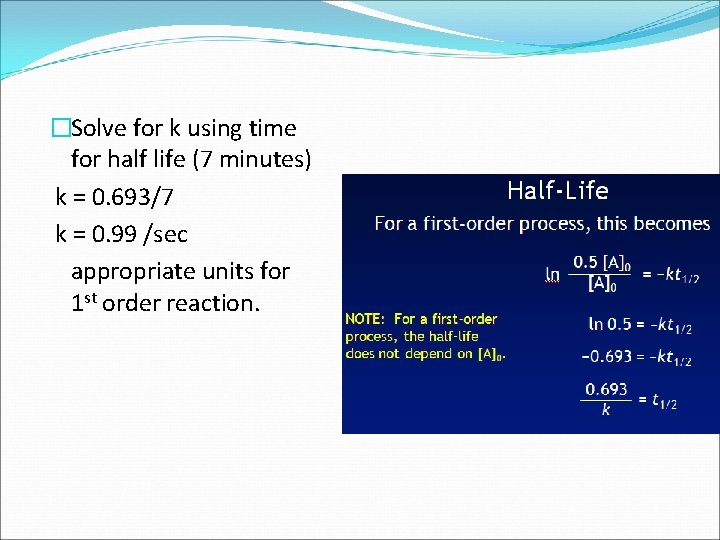
�Solve for k using time for half life (7 minutes) k = 0. 693/7 k = 0. 99 /sec appropriate units for 1 st order reaction.
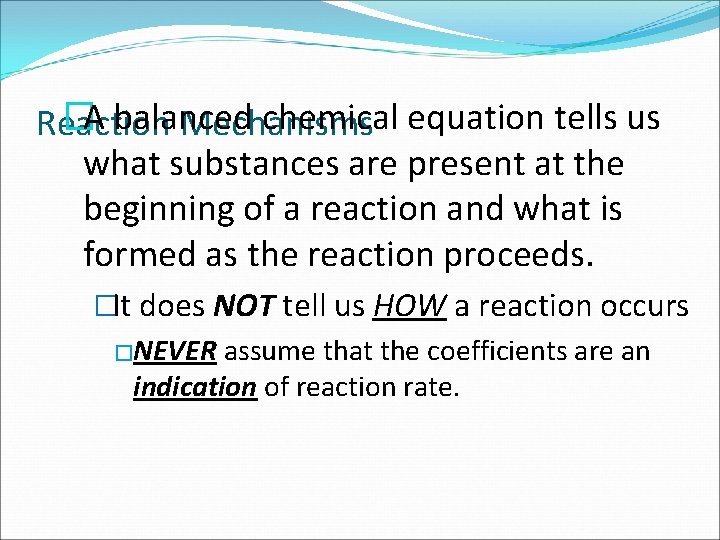
�A balanced chemical equation tells us Reaction Mechanisms what substances are present at the beginning of a reaction and what is formed as the reaction proceeds. �It does NOT tell us HOW a reaction occurs �NEVER assume that the coefficients are an indication of reaction rate.
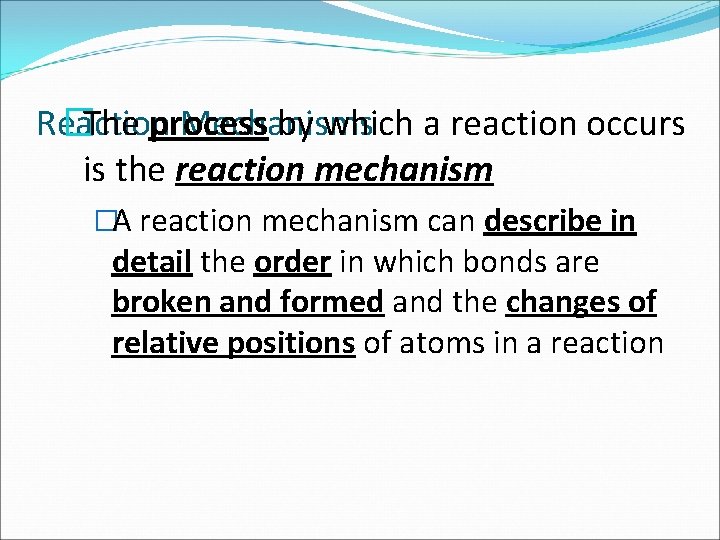
�The process by which a reaction occurs Reaction Mechanisms is the reaction mechanism �A reaction mechanism can describe in detail the order in which bonds are broken and formed and the changes of relative positions of atoms in a reaction
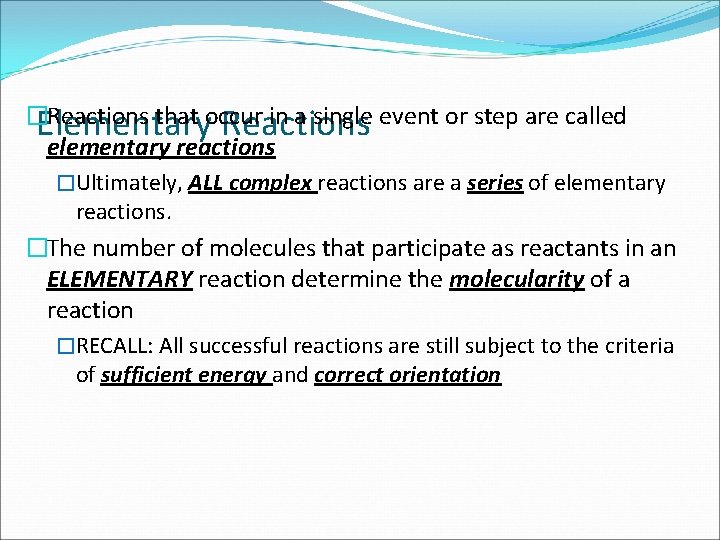
� Reactions that occur in a single event or step are called Elementary Reactions elementary reactions �Ultimately, ALL complex reactions are a series of elementary reactions. �The number of molecules that participate as reactants in an ELEMENTARY reaction determine the molecularity of a reaction �RECALL: All successful reactions are still subject to the criteria of sufficient energy and correct orientation
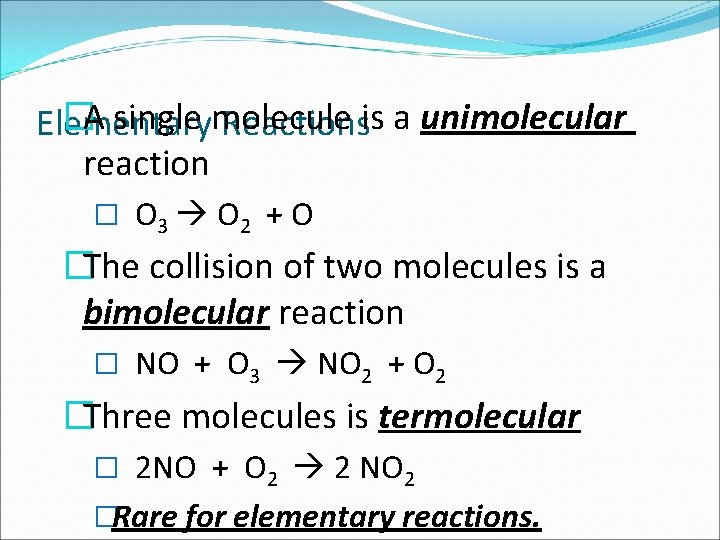
�A single molecule Elementary Reactionsis a unimolecular reaction � O 3 O 2 + O �The collision of two molecules is a bimolecular reaction � NO + O 3 NO 2 + O 2 �Three molecules is termolecular � 2 NO + O 2 2 NO 2 �Rare for elementary reactions.
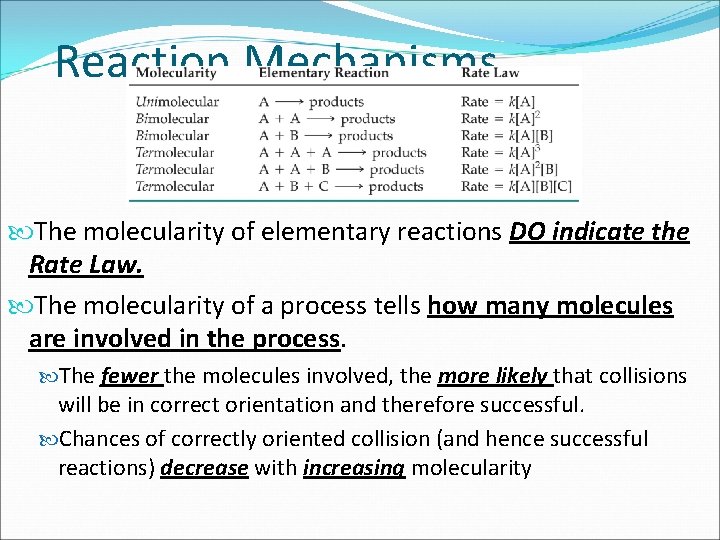
Reaction Mechanisms The molecularity of elementary reactions DO indicate the Rate Law. The molecularity of a process tells how many molecules are involved in the process. The fewer the molecules involved, the more likely that collisions will be in correct orientation and therefore successful. Chances of correctly oriented collision (and hence successful reactions) decrease with increasing molecularity
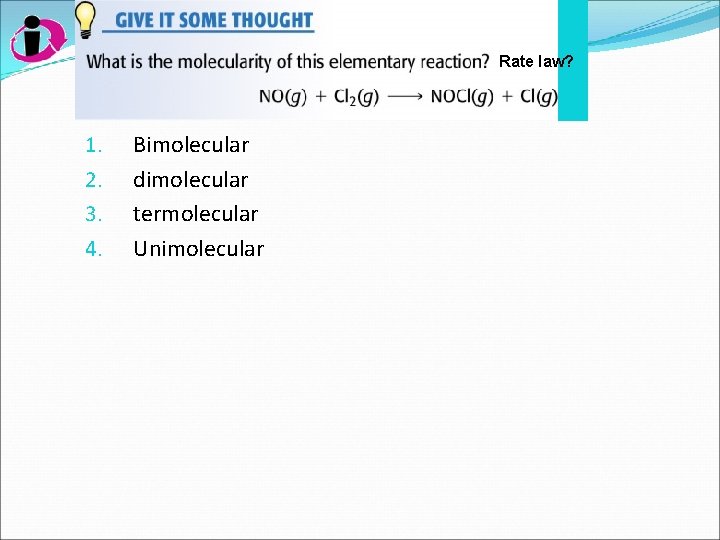
Rate law? 1. 2. 3. 4. Bimolecular dimolecular termolecular Unimolecular
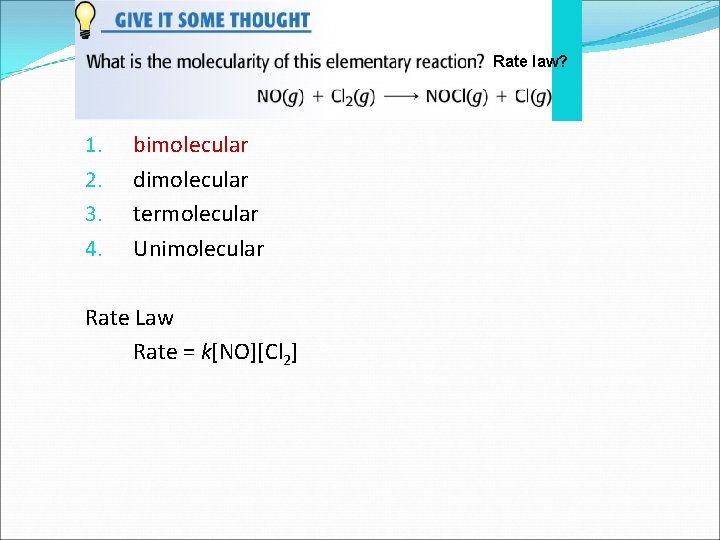
Rate law? 1. 2. 3. 4. bimolecular dimolecular termolecular Unimolecular Rate Law Rate = k[NO][Cl 2]
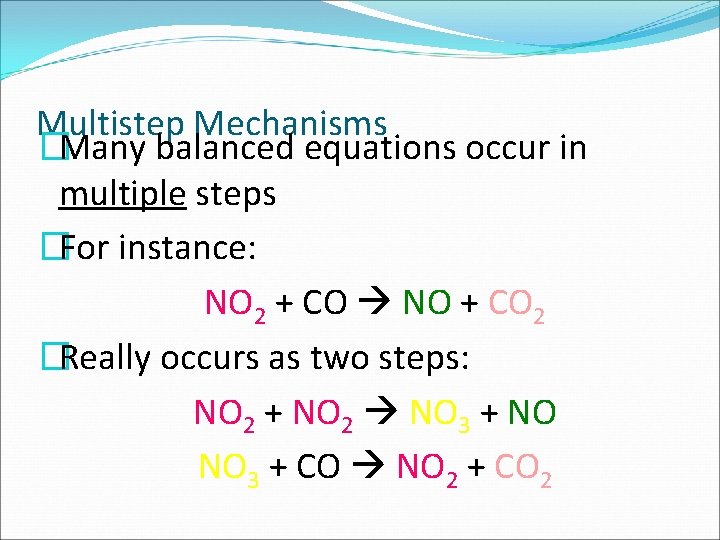
Multistep Mechanisms �Many balanced equations occur in multiple steps �For instance: NO 2 + CO NO + CO 2 �Really occurs as two steps: NO 2 + NO 2 NO 3 + NO NO 3 + CO NO 2 + CO 2
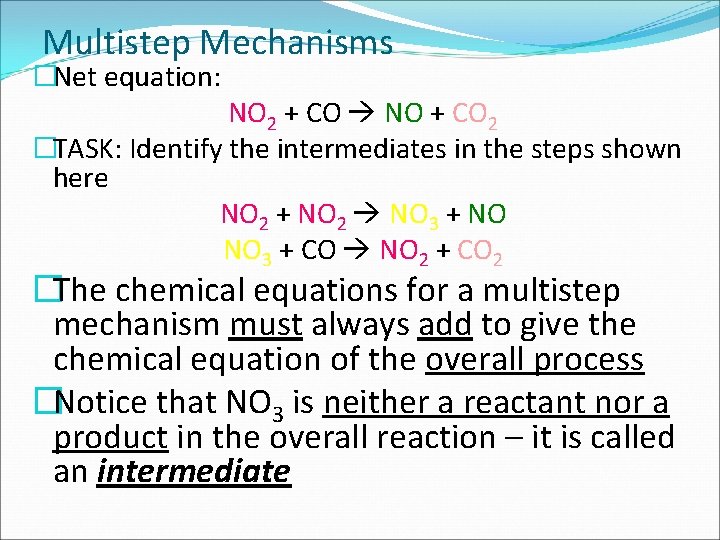
Multistep Mechanisms �Net equation: NO 2 + CO NO + CO 2 �TASK: Identify the intermediates in the steps shown here NO 2 + NO 2 NO 3 + NO NO 3 + CO NO 2 + CO 2 �The chemical equations for a multistep mechanism must always add to give the chemical equation of the overall process �Notice that NO 3 is neither a reactant nor a product in the overall reaction – it is called an intermediate
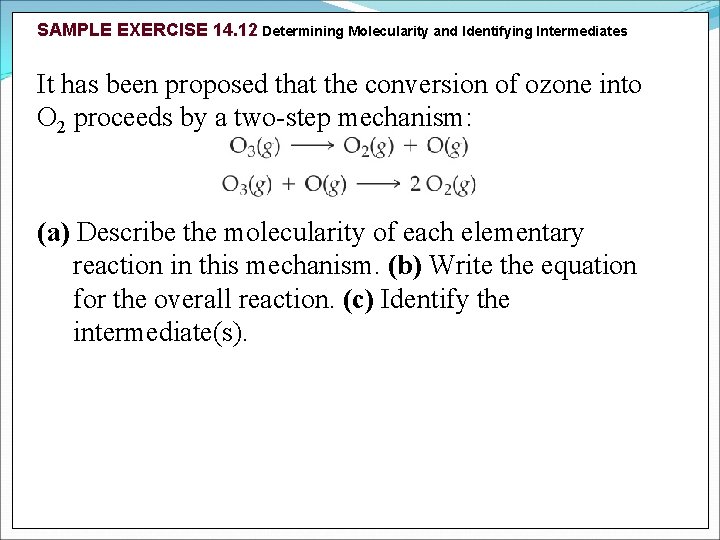
SAMPLE EXERCISE 14. 12 Determining Molecularity and Identifying Intermediates It has been proposed that the conversion of ozone into O 2 proceeds by a two-step mechanism: (a) Describe the molecularity of each elementary reaction in this mechanism. (b) Write the equation for the overall reaction. (c) Identify the intermediate(s).
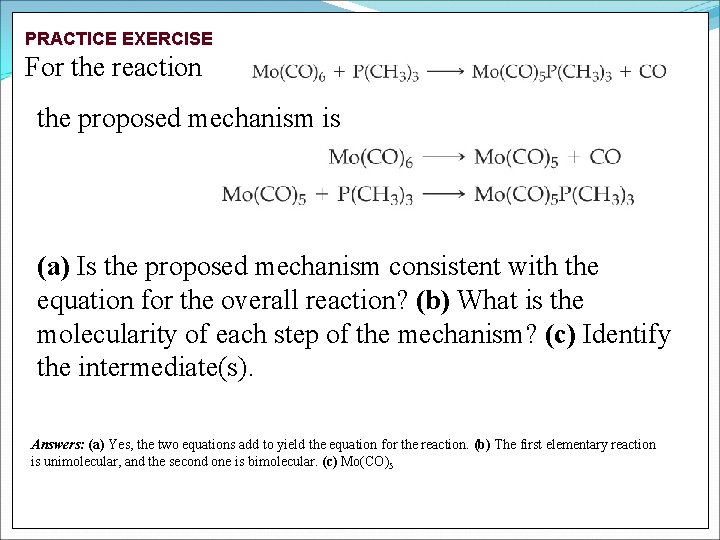
PRACTICE EXERCISE For the reaction the proposed mechanism is (a) Is the proposed mechanism consistent with the equation for the overall reaction? (b) What is the molecularity of each step of the mechanism? (c) Identify the intermediate(s). Answers: (a) Yes, the two equations add to yield the equation for the reaction. (b) The first elementary reaction is unimolecular, and the second one is bimolecular. (c) Mo(CO)5
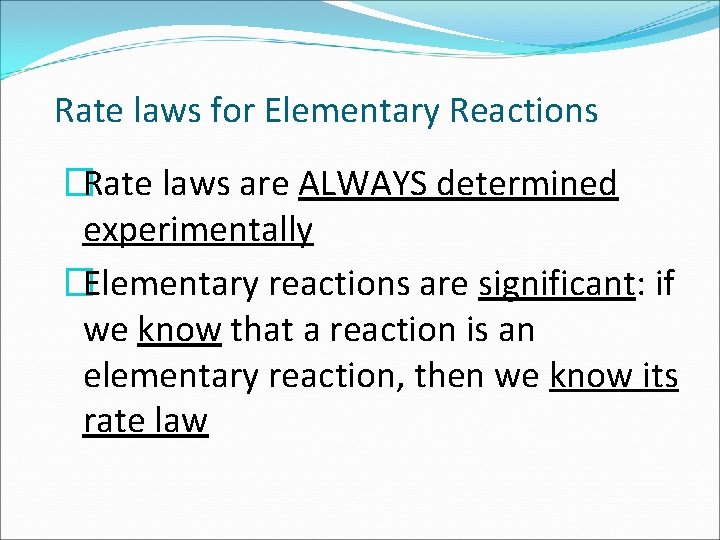
Rate laws for Elementary Reactions �Rate laws are ALWAYS determined experimentally �Elementary reactions are significant: if we know that a reaction is an elementary reaction, then we know its rate law
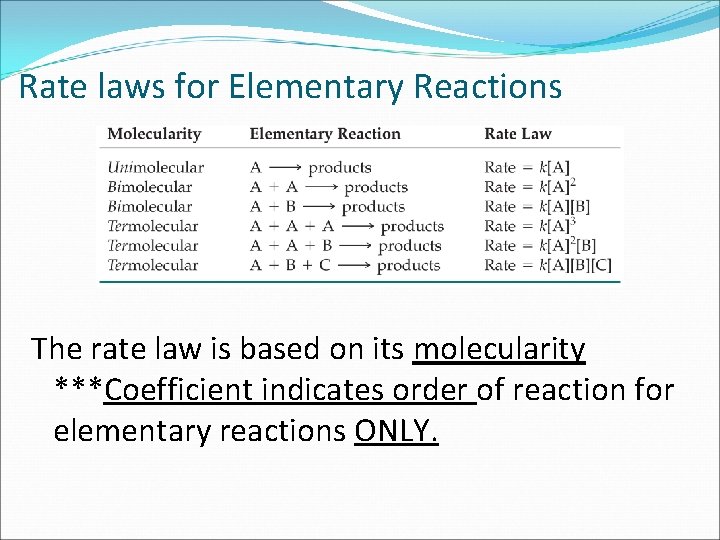
Rate laws for Elementary Reactions The rate law is based on its molecularity ***Coefficient indicates order of reaction for elementary reactions ONLY.
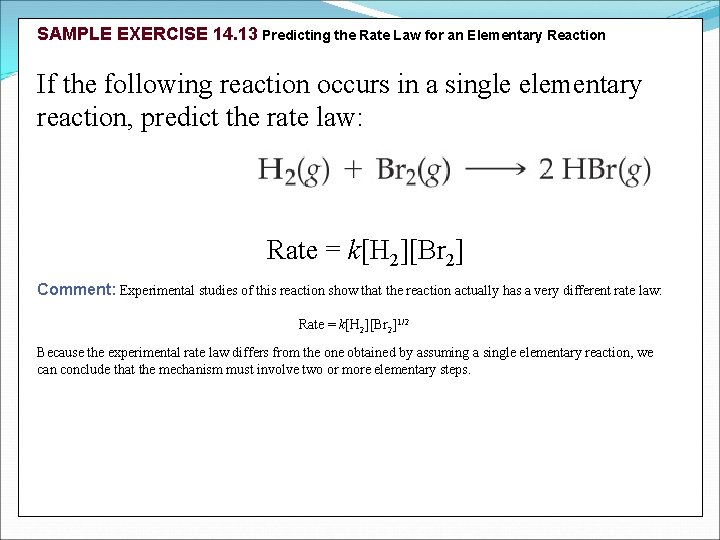
SAMPLE EXERCISE 14. 13 Predicting the Rate Law for an Elementary Reaction If the following reaction occurs in a single elementary reaction, predict the rate law: Rate = k[H 2][Br 2] Comment: Experimental studies of this reaction show that the reaction actually has a very different rate law: Rate = k[H 2][Br 2]1/2 Because the experimental rate law differs from the one obtained by assuming a single elementary reaction, we can conclude that the mechanism must involve two or more elementary steps.
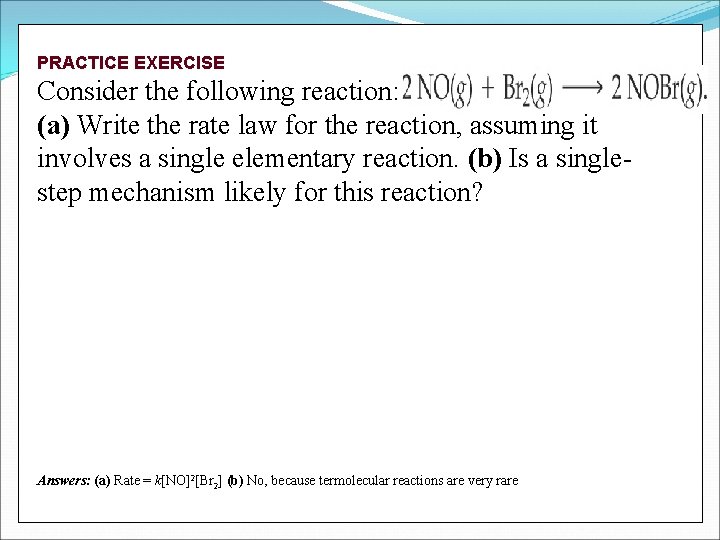
PRACTICE EXERCISE Consider the following reaction: (a) Write the rate law for the reaction, assuming it involves a single elementary reaction. (b) Is a singlestep mechanism likely for this reaction? Answers: (a) Rate = k[NO]2[Br 2] (b) No, because termolecular reactions are very rare
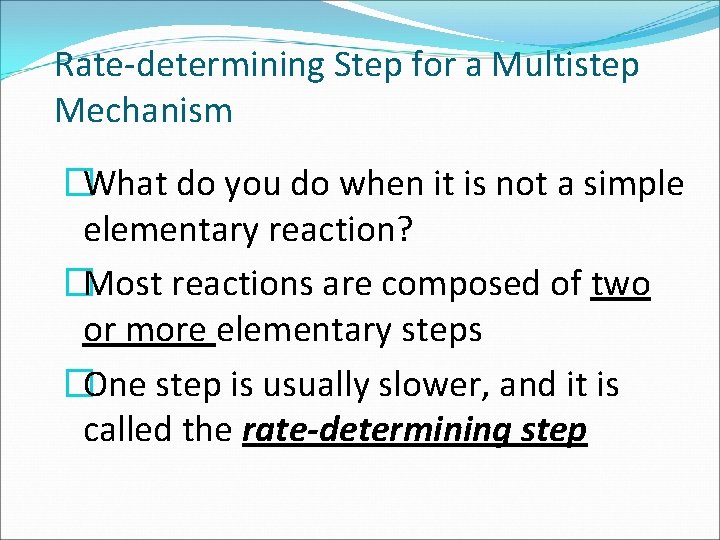
Rate-determining Step for a Multistep Mechanism �What do you do when it is not a simple elementary reaction? �Most reactions are composed of two or more elementary steps �One step is usually slower, and it is called the rate-determining step
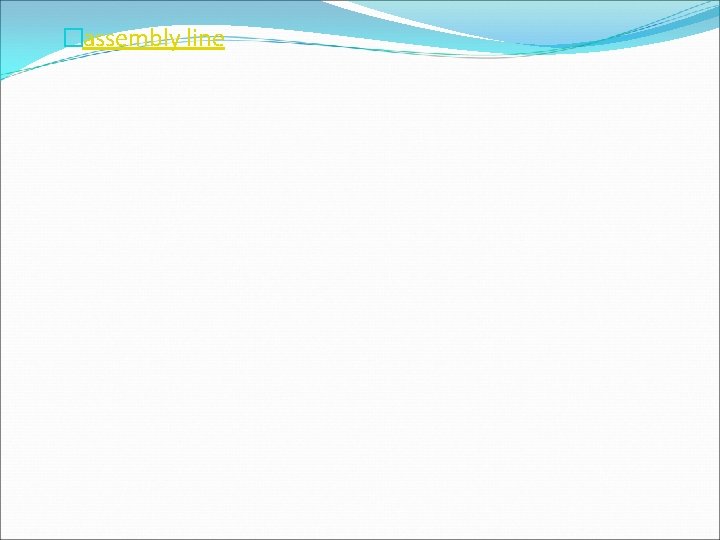
�assembly line
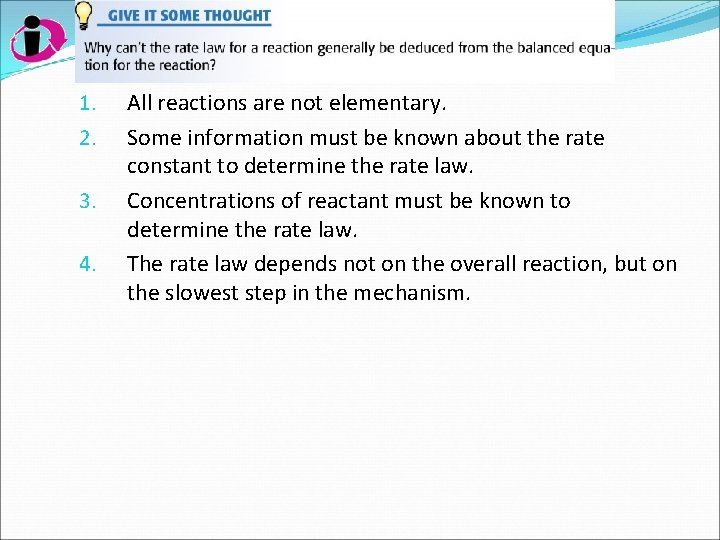
1. 2. 3. 4. All reactions are not elementary. Some information must be known about the rate constant to determine the rate law. Concentrations of reactant must be known to determine the rate law. The rate law depends not on the overall reaction, but on the slowest step in the mechanism.
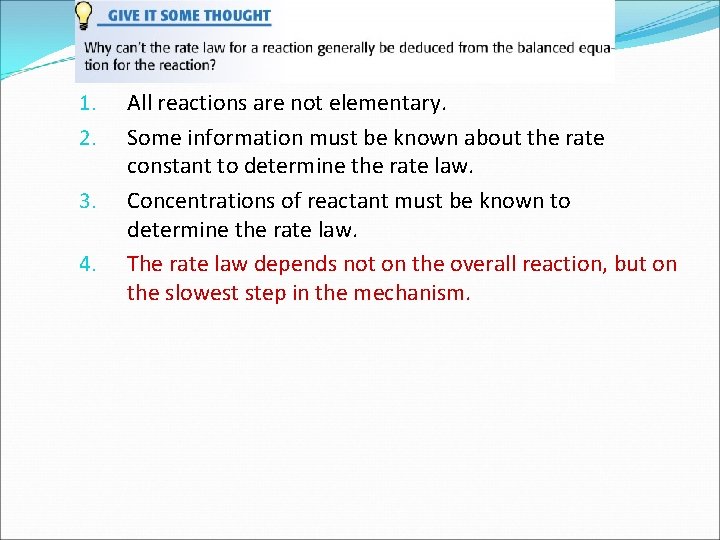
1. 2. 3. 4. All reactions are not elementary. Some information must be known about the rate constant to determine the rate law. Concentrations of reactant must be known to determine the rate law. The rate law depends not on the overall reaction, but on the slowest step in the mechanism.
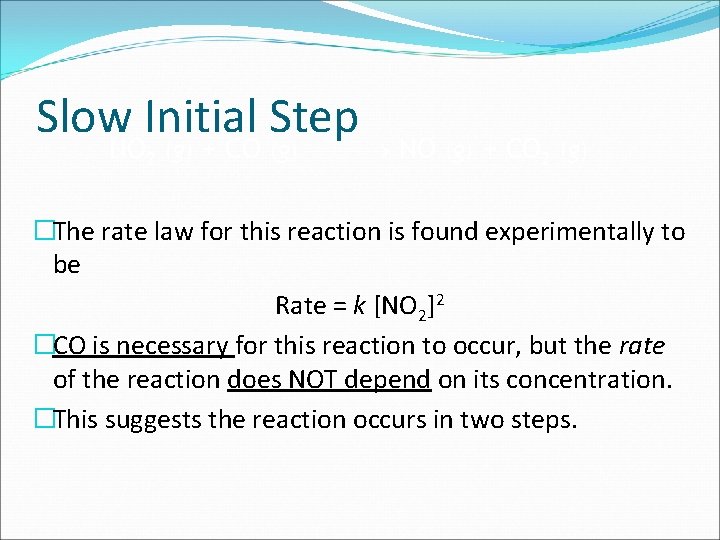
Slow Initial Step NO 2 (g) + CO (g) NO (g) + CO 2 (g) �The rate law for this reaction is found experimentally to be Rate = k [NO 2]2 �CO is necessary for this reaction to occur, but the rate of the reaction does NOT depend on its concentration. �This suggests the reaction occurs in two steps.
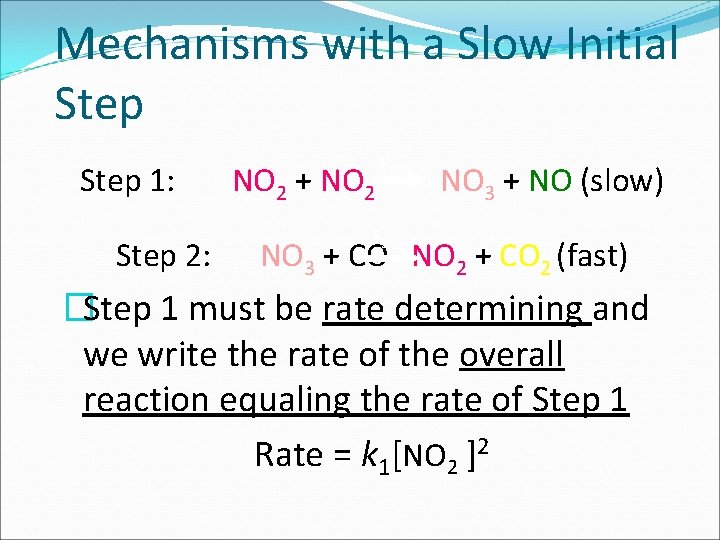
Mechanisms with a Slow Initial Step 1: Step 2: NO 2 + NO 2 k 1 NO 3 + NO (slow) k 2 NO 3 + CO NO 2 + CO 2 (fast) �Step 1 must be rate determining and we write the rate of the overall reaction equaling the rate of Step 1 Rate = k 1[NO 2 ]2
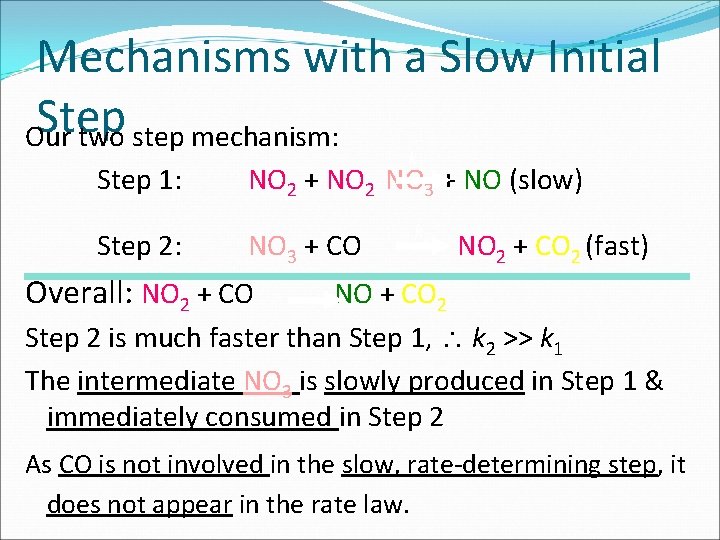
Mechanisms with a Slow Initial Step Our two step mechanism: Step 1: Step 2: k 1 NO 2 + NO 2 NO 3 + NO (slow) NO 3 + CO k 2 NO 2 + CO 2 (fast) Overall: NO 2 + CO NO + CO 2 Step 2 is much faster than Step 1, k 2 >> k 1 The intermediate NO 3 is slowly produced in Step 1 & immediately consumed in Step 2 As CO is not involved in the slow, rate-determining step, it does not appear in the rate law.
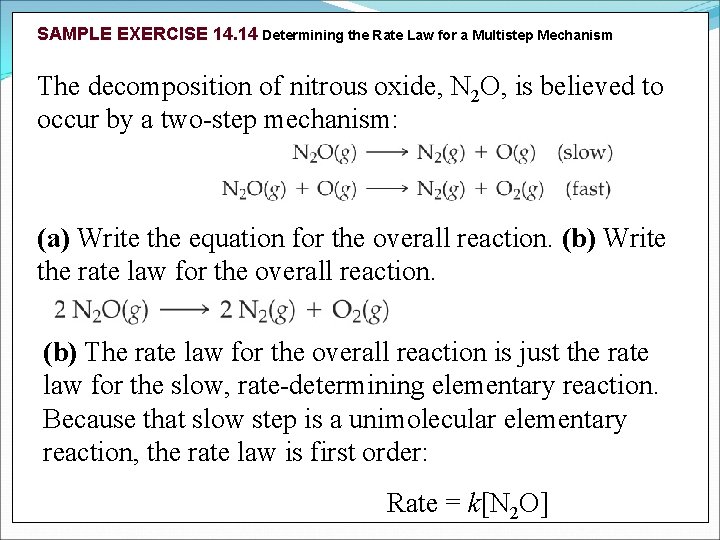
SAMPLE EXERCISE 14. 14 Determining the Rate Law for a Multistep Mechanism The decomposition of nitrous oxide, N 2 O, is believed to occur by a two-step mechanism: (a) Write the equation for the overall reaction. (b) Write the rate law for the overall reaction. (b) The rate law for the overall reaction is just the rate law for the slow, rate-determining elementary reaction. Because that slow step is a unimolecular elementary reaction, the rate law is first order: Rate = k[N 2 O]
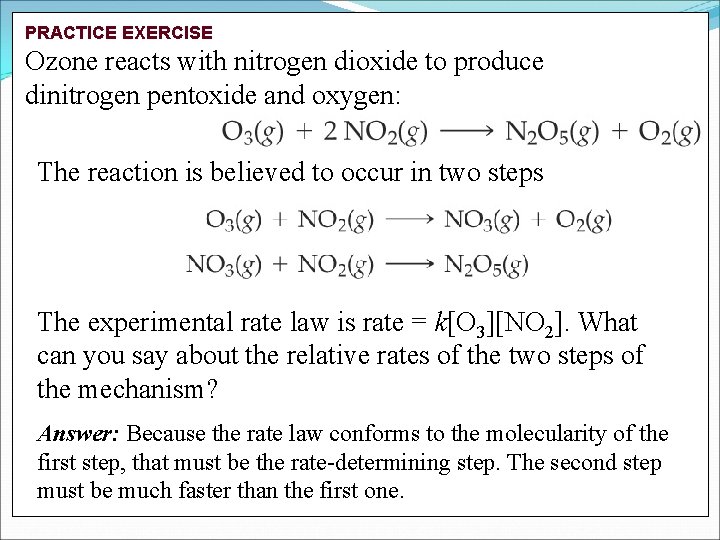
PRACTICE EXERCISE Ozone reacts with nitrogen dioxide to produce dinitrogen pentoxide and oxygen: The reaction is believed to occur in two steps The experimental rate law is rate = k[O 3][NO 2]. What can you say about the relative rates of the two steps of the mechanism? Answer: Because the rate law conforms to the molecularity of the first step, that must be the rate-determining step. The second step must be much faster than the first one.
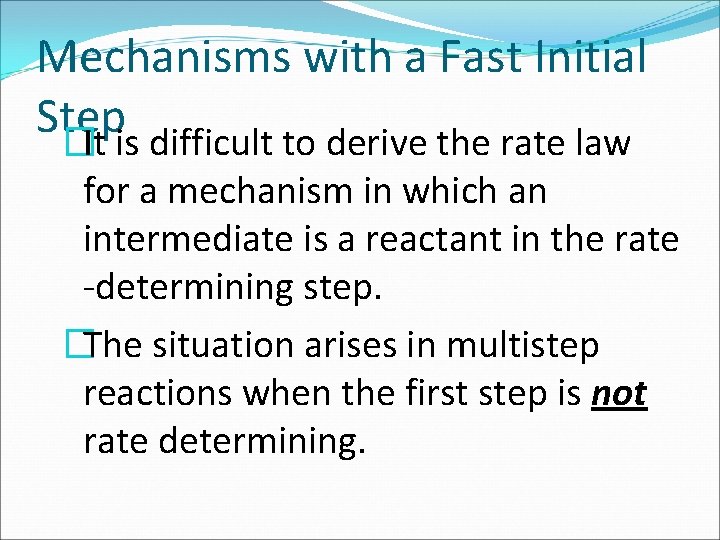
Mechanisms with a Fast Initial Step �It is difficult to derive the rate law for a mechanism in which an intermediate is a reactant in the rate -determining step. �The situation arises in multistep reactions when the first step is not rate determining.
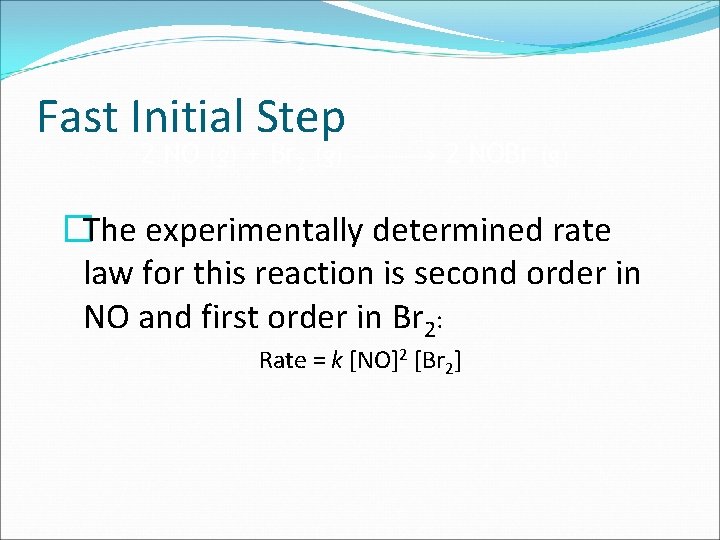
Fast Initial Step 2 NO (g) + Br 2 (g) 2 NOBr (g) �The experimentally determined rate law for this reaction is second order in NO and first order in Br 2: Rate = k [NO]2 [Br 2]
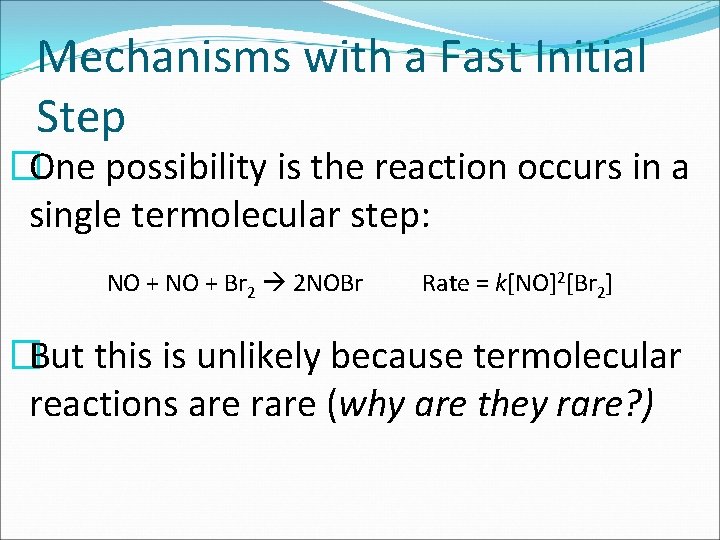
Mechanisms with a Fast Initial Step �One possibility is the reaction occurs in a single termolecular step: NO + Br 2 2 NOBr Rate = k[NO]2[Br 2] �But this is unlikely because termolecular reactions are rare (why are they rare? )
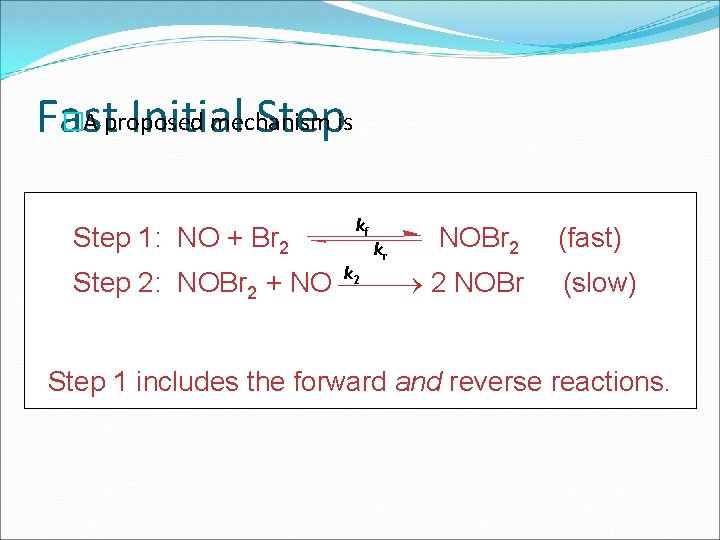
�A proposed mechanism Fast Initial Stepis Step 1: NO + Br 2 kf kr NOBr 2 k 2 Step 2: NOBr 2 + NO 2 NOBr (fast) (slow) Step 1 includes the forward and reverse reactions.
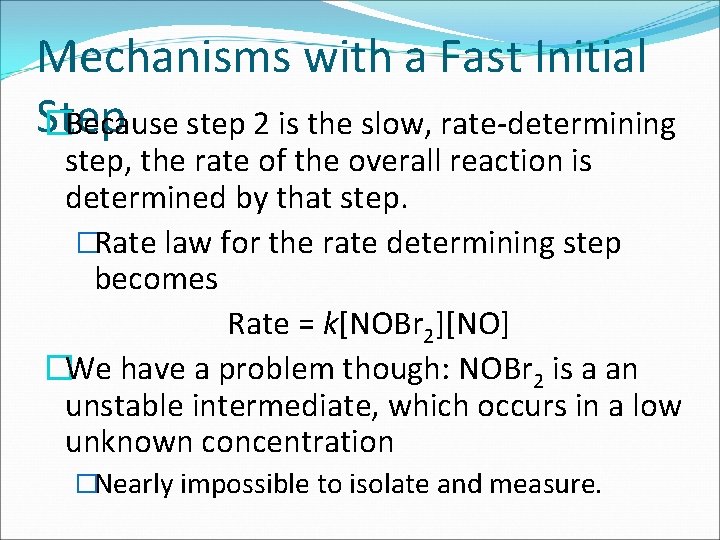
Mechanisms with a Fast Initial Step �Because step 2 is the slow, rate-determining step, the rate of the overall reaction is determined by that step. �Rate law for the rate determining step becomes Rate = k[NOBr 2][NO] �We have a problem though: NOBr 2 is a an unstable intermediate, which occurs in a low unknown concentration �Nearly impossible to isolate and measure.
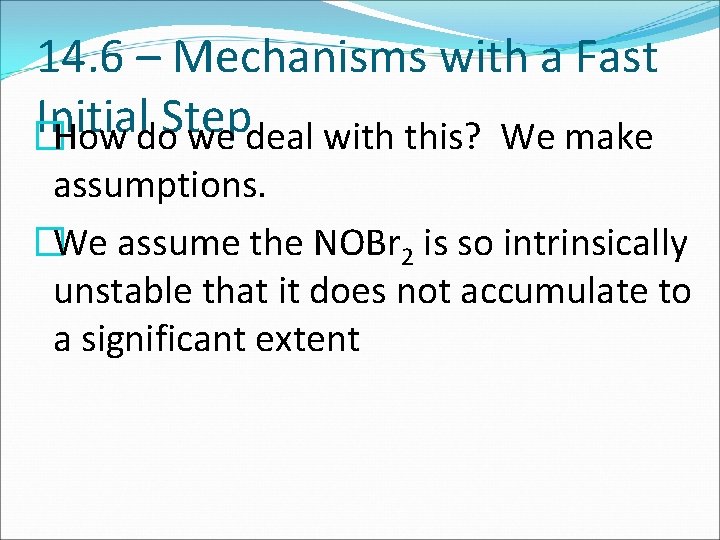
14. 6 – Mechanisms with a Fast Initial Step �How do we deal with this? We make assumptions. �We assume the NOBr 2 is so intrinsically unstable that it does not accumulate to a significant extent
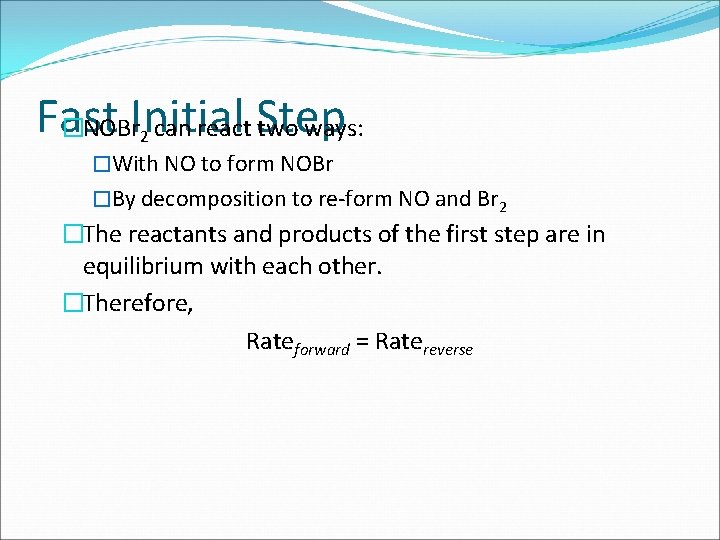
Fast �NOBr. Initial can react Step two ways: 2 �With NO to form NOBr �By decomposition to re-form NO and Br 2 �The reactants and products of the first step are in equilibrium with each other. �Therefore, Rateforward = Ratereverse
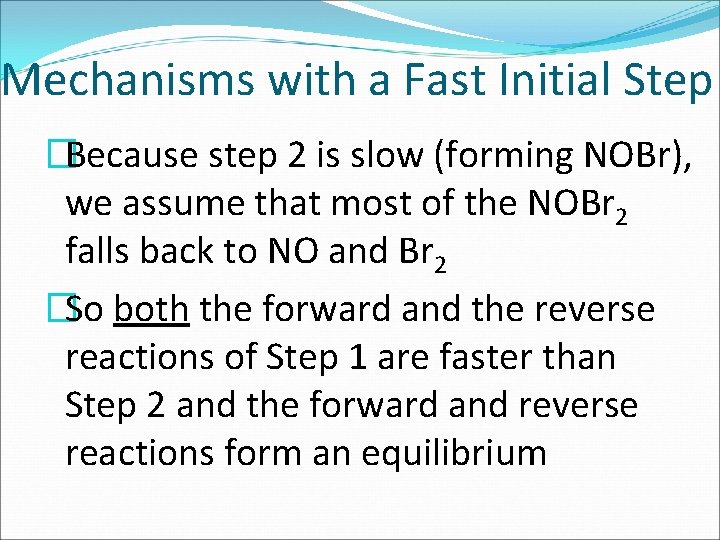
Mechanisms with a Fast Initial Step �Because step 2 is slow (forming NOBr), we assume that most of the NOBr 2 falls back to NO and Br 2 �So both the forward and the reverse reactions of Step 1 are faster than Step 2 and the forward and reverse reactions form an equilibrium
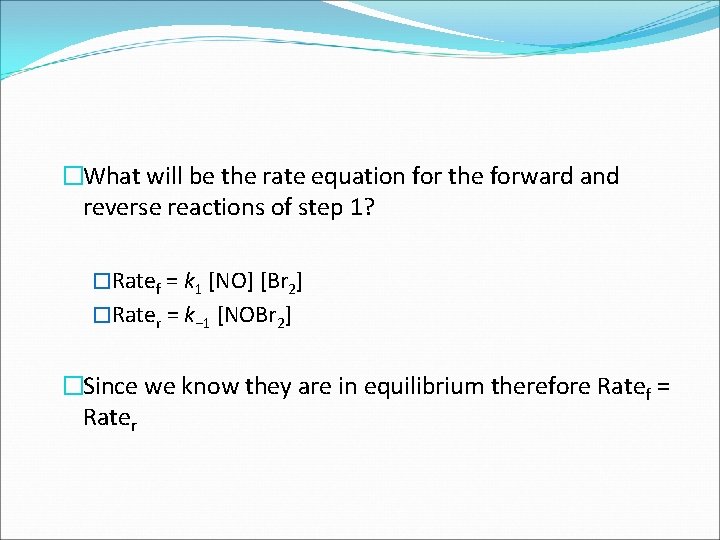
�What will be the rate equation for the forward and reverse reactions of step 1? �Ratef = k 1 [NO] [Br 2] �Rater = k− 1 [NOBr 2] �Since we know they are in equilibrium therefore Ratef = Rater
![Fast Initial Step Because Ratef Rater k 1 NO Br 2 Fast Initial Step �Because Ratef = Rater , k 1 [NO] [Br 2] =](https://slidetodoc.com/presentation_image_h/1c9abc1fe8d10b8ee4aadf33e9ca8558/image-136.jpg)
Fast Initial Step �Because Ratef = Rater , k 1 [NO] [Br 2] = k− 1 [NOBr 2] �Solving for [NOBr 2] gives us k 1 [NO] [Br ] = [NOBr ] − 2 2 k 1
![Substituting this Step expression for NOBr in the rate law Fast Initial for Substituting this Step expression for [NOBr ] in the rate law Fast Initial for](https://slidetodoc.com/presentation_image_h/1c9abc1fe8d10b8ee4aadf33e9ca8558/image-137.jpg)
Substituting this Step expression for [NOBr ] in the rate law Fast Initial for the rate-determining step gives Rate = 2 k 2 k 1 [NO] [Br 2] [NO] − k 1 = k [NO]2 [Br 2] Where k is equal to k 2(k 1/k 1 -)
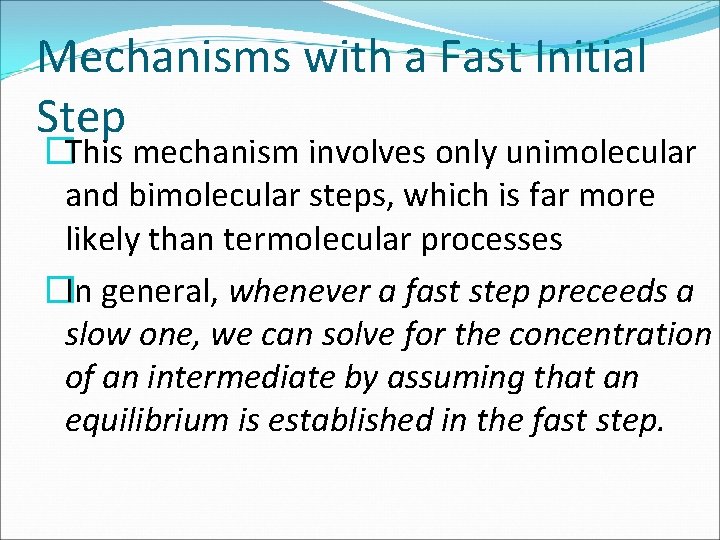
Mechanisms with a Fast Initial Step �This mechanism involves only unimolecular and bimolecular steps, which is far more likely than termolecular processes �In general, whenever a fast step preceeds a slow one, we can solve for the concentration of an intermediate by assuming that an equilibrium is established in the fast step.
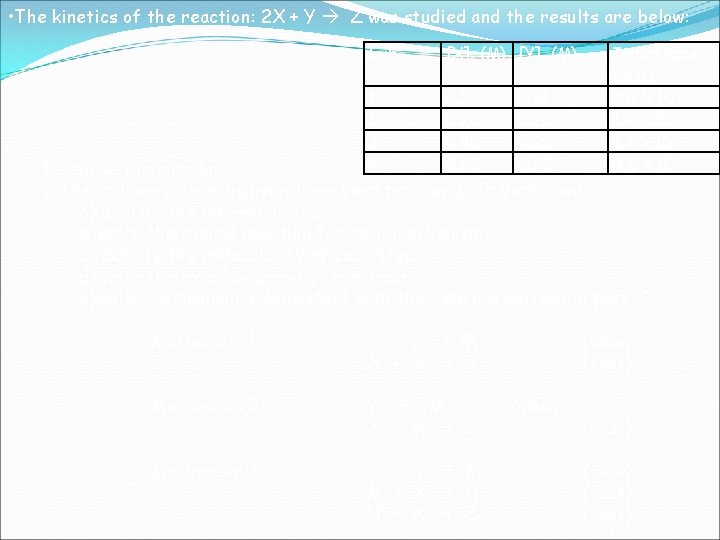
• The kinetics of the reaction: 2 X + Y Z was studied and the results are below: Exp [X]o (M) [Y]o (M) 1 2 3 4 0. 20 0. 40 0. 60 0. 10 0. 20 0. 60 Initial Rate (M/s) 7. 0 X 10 -4 1. 4 X 10 -3 4. 2 X 10 -3 1. Deduce the rate law. 2. The following 3 mechanisms have been proposed. In each case, a)identify the intermediates b)write the overall reaction for each mechanism c)identify the molecularity of each step d)write the rate law derived from each e)which mechanism is consistent with the rate law derived in part a? Mechanism 1: X + Y M X + M Z Mechanism 2: Y M X + M Z Mechanism 3: Y M M + X N N + X Z (slow) (fast)
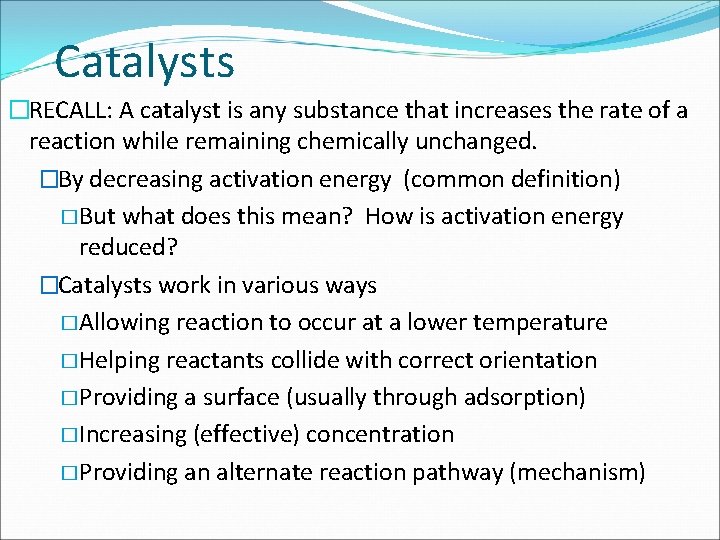
Catalysts �RECALL: A catalyst is any substance that increases the rate of a reaction while remaining chemically unchanged. �By decreasing activation energy (common definition) � But what does this mean? How is activation energy reduced? �Catalysts work in various ways � Allowing reaction to occur at a lower temperature � Helping reactants collide with correct orientation � Providing a surface (usually through adsorption) � Increasing (effective) concentration � Providing an alternate reaction pathway (mechanism)
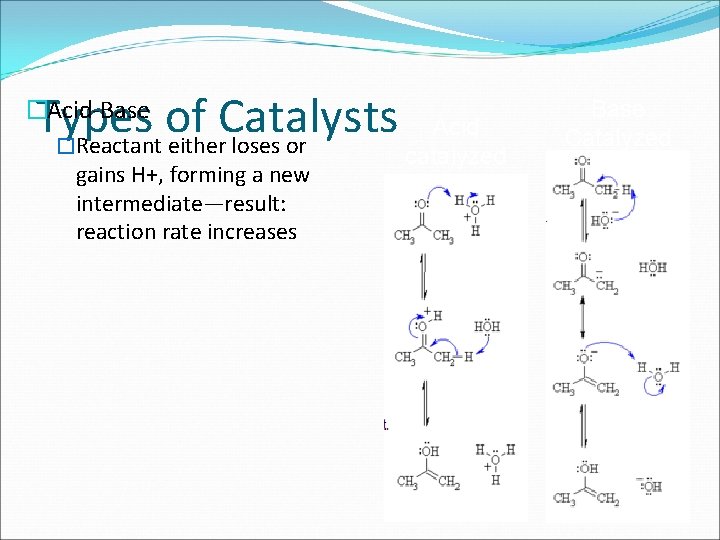
Types of Catalysts �Acid-Base �Reactant either loses or gains H+, forming a new intermediate—result: reaction rate increases Acid catalyzed Base Catalyzed
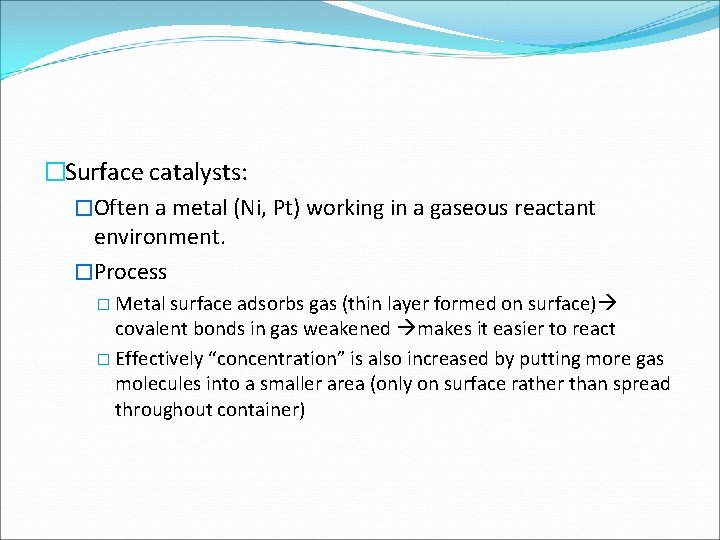
�Surface catalysts: �Often a metal (Ni, Pt) working in a gaseous reactant environment. �Process � Metal surface adsorbs gas (thin layer formed on surface) covalent bonds in gas weakened makes it easier to react � Effectively “concentration” is also increased by putting more gas molecules into a smaller area (only on surface rather than spread throughout container)
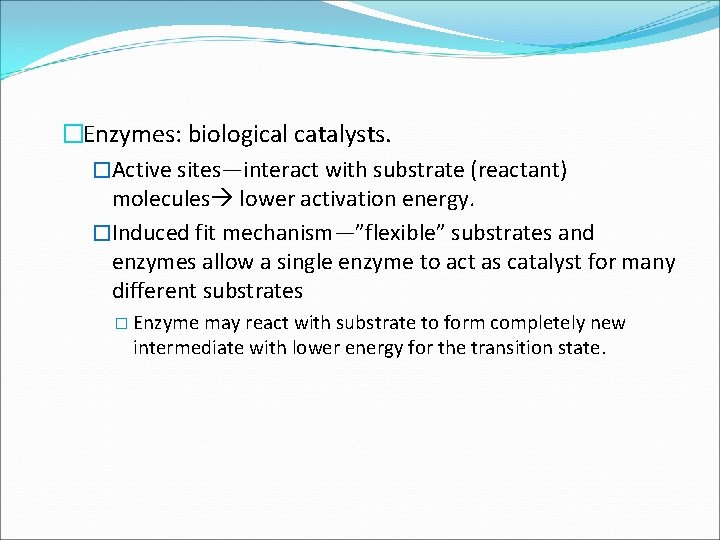
�Enzymes: biological catalysts. �Active sites—interact with substrate (reactant) molecules lower activation energy. �Induced fit mechanism—”flexible” substrates and enzymes allow a single enzyme to act as catalyst for many different substrates � Enzyme may react with substrate to form completely new intermediate with lower energy for the transition state.
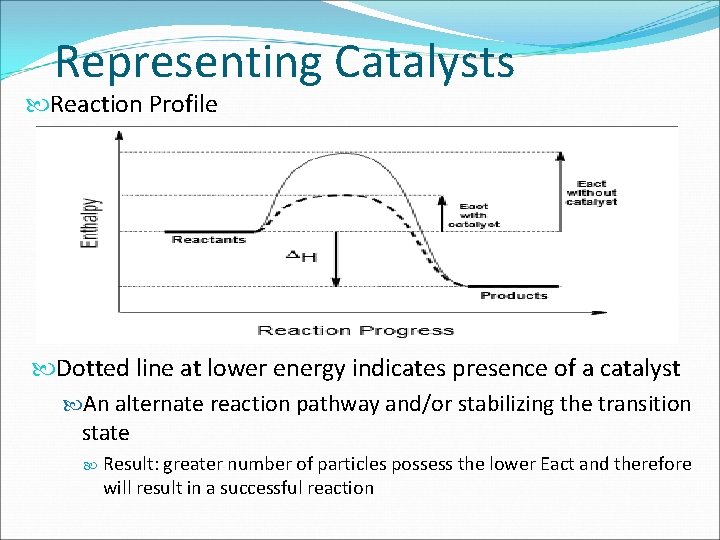
Representing Catalysts Reaction Profile Dotted line at lower energy indicates presence of a catalyst An alternate reaction pathway and/or stabilizing the transition state Result: greater number of particles possess the lower Eact and therefore will result in a successful reaction
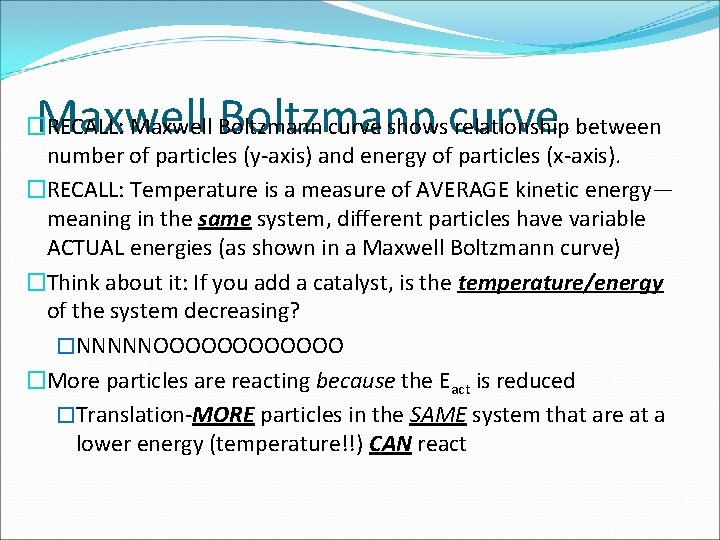
Maxwell Boltzmann curve �RECALL: Maxwell Boltzmann curve shows relationship between number of particles (y-axis) and energy of particles (x-axis). �RECALL: Temperature is a measure of AVERAGE kinetic energy— meaning in the same system, different particles have variable ACTUAL energies (as shown in a Maxwell Boltzmann curve) �Think about it: If you add a catalyst, is the temperature/energy of the system decreasing? �NNNNNOOOOOO �More particles are reacting because the Eact is reduced �Translation-MORE particles in the SAME system that are at a lower energy (temperature!!) CAN react
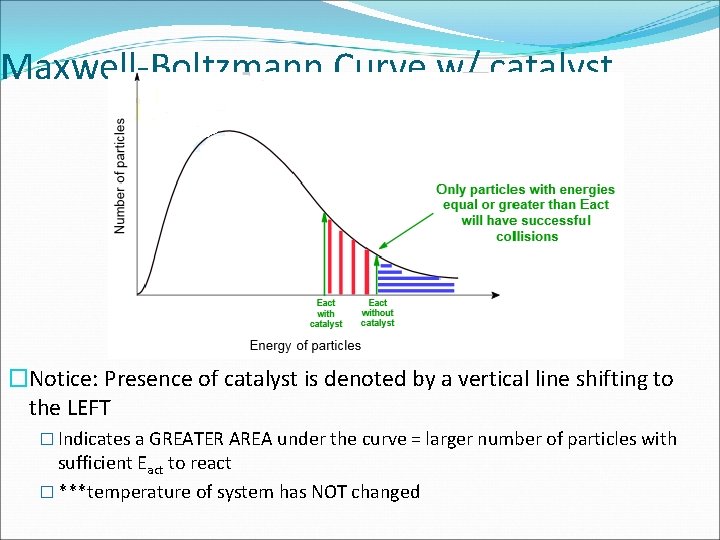
Maxwell-Boltzmann Curve w/ catalyst �Notice: Presence of catalyst is denoted by a vertical line shifting to the LEFT � Indicates a GREATER AREA under the curve = larger number of particles with sufficient Eact to react � ***temperature of system has NOT changed
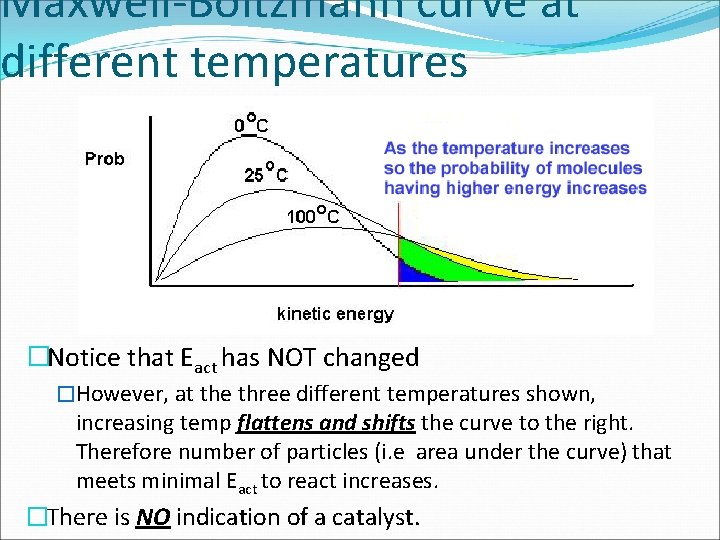
Maxwell-Boltzmann curve at different temperatures �Notice that Eact has NOT changed �However, at the three different temperatures shown, increasing temp flattens and shifts the curve to the right. Therefore number of particles (i. e area under the curve) that meets minimal Eact to react increases. �There is NO indication of a catalyst.
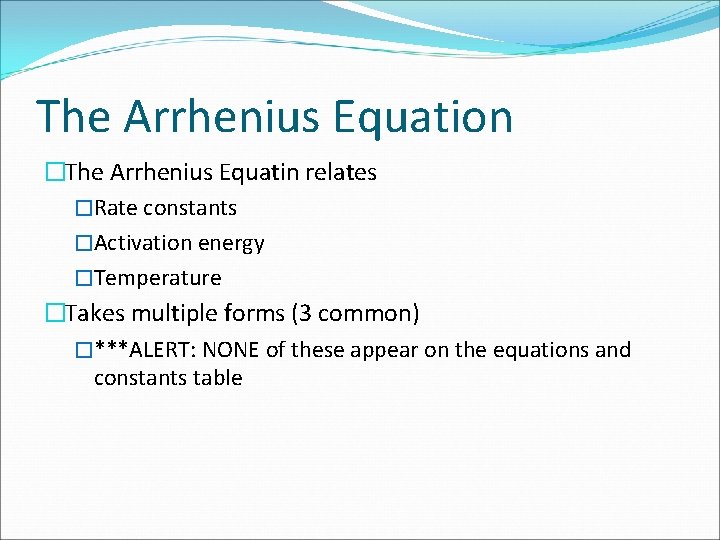
The Arrhenius Equation �The Arrhenius Equatin relates �Rate constants �Activation energy �Temperature �Takes multiple forms (3 common) �***ALERT: NONE of these appear on the equations and constants table
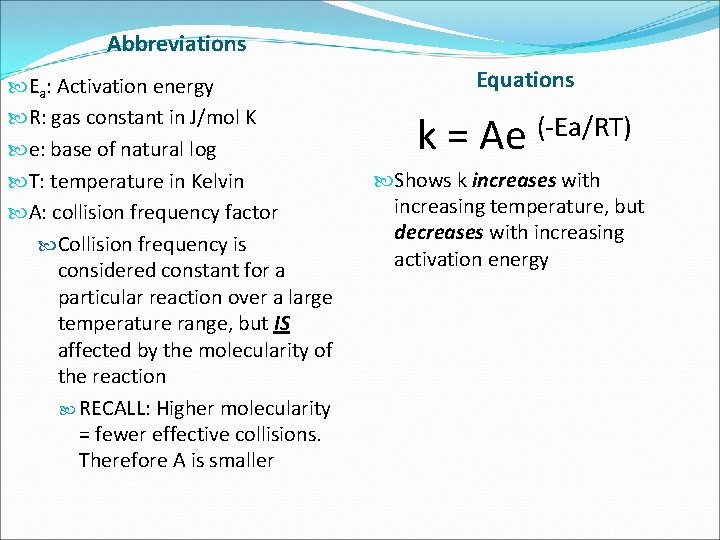
Abbreviations Ea: Activation energy R: gas constant in J/mol K e: base of natural log T: temperature in Kelvin A: collision frequency factor Collision frequency is considered constant for a particular reaction over a large temperature range, but IS affected by the molecularity of the reaction RECALL: Higher molecularity = fewer effective collisions. Therefore A is smaller Equations k = Ae (-Ea/RT) Shows k increases with increasing temperature, but decreases with increasing activation energy
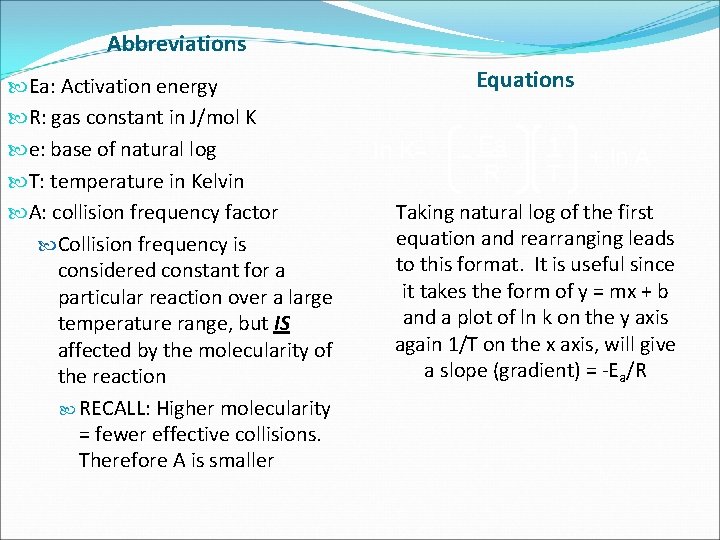
Abbreviations Ea: Activation energy R: gas constant in J/mol K e: base of natural log T: temperature in Kelvin A: collision frequency factor Collision frequency is considered constant for a particular reaction over a large temperature range, but IS affected by the molecularity of the reaction RECALL: Higher molecularity = fewer effective collisions. Therefore A is smaller Equations ln K= _ Ea R 1 T + ln A Taking natural log of the first equation and rearranging leads to this format. It is useful since it takes the form of y = mx + b and a plot of ln k on the y axis again 1/T on the x axis, will give a slope (gradient) = -Ea/R
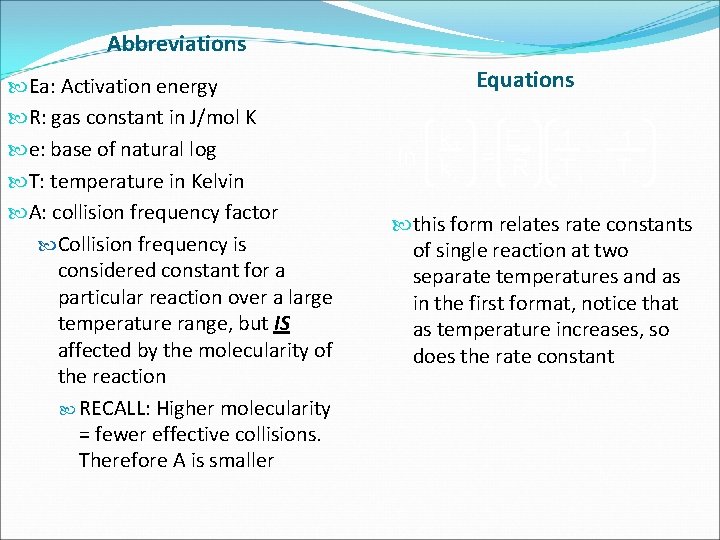
Abbreviations Ea: Activation energy R: gas constant in J/mol K e: base of natural log T: temperature in Kelvin A: collision frequency factor Collision frequency is considered constant for a particular reaction over a large temperature range, but IS affected by the molecularity of the reaction RECALL: Higher molecularity = fewer effective collisions. Therefore A is smaller Equations k 1 Ea ln k = R 2 1 _ 1_ T 1 T 2 this form relates rate constants of single reaction at two separate temperatures and as in the first format, notice that as temperature increases, so does the rate constant
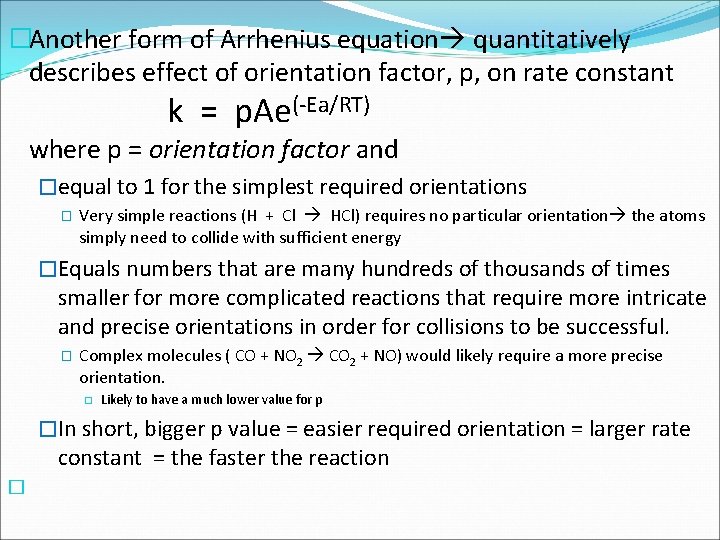
�Another form of Arrhenius equation quantitatively describes effect of orientation factor, p, on rate constant k = p. Ae(-Ea/RT) where p = orientation factor and �equal to 1 for the simplest required orientations � Very simple reactions (H + Cl HCl) requires no particular orientation the atoms simply need to collide with sufficient energy �Equals numbers that are many hundreds of thousands of times smaller for more complicated reactions that require more intricate and precise orientations in order for collisions to be successful. � Complex molecules ( CO + NO 2 CO 2 + NO) would likely require a more precise orientation. � Likely to have a much lower value for p �In short, bigger p value = easier required orientation = larger rate constant = the faster the reaction �
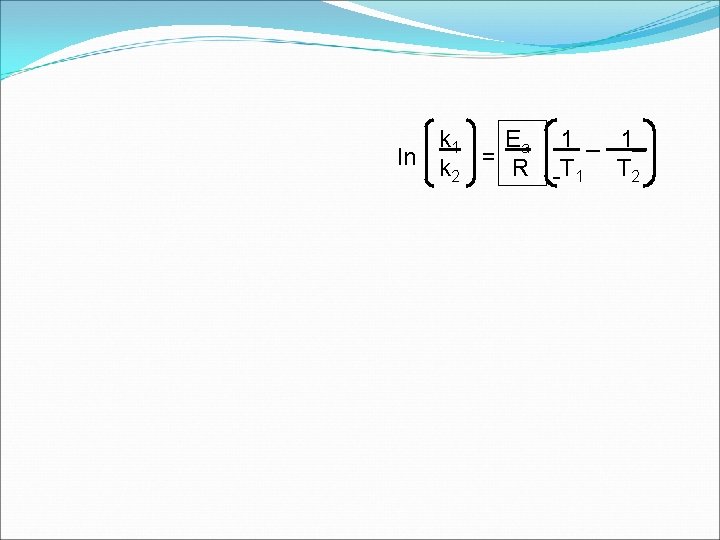
k 1 Ea ln k = R 2 1 _ 1_ T 1 T 2
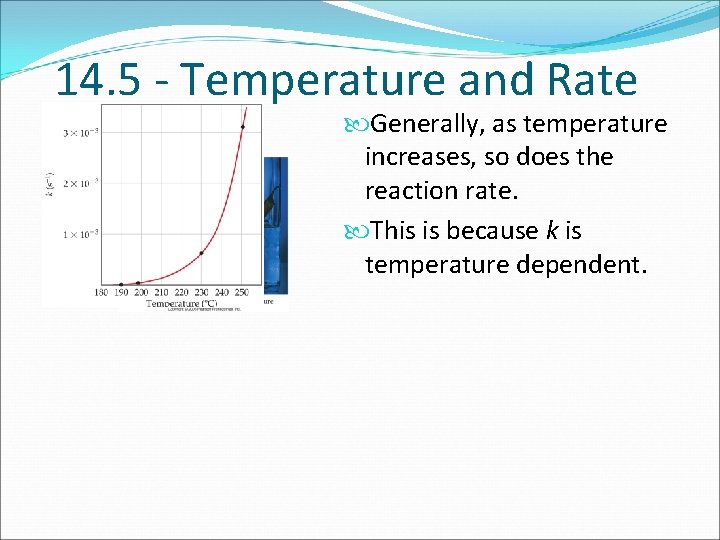
14. 5 - Temperature and Rate Generally, as temperature increases, so does the reaction rate. This is because k is temperature dependent.
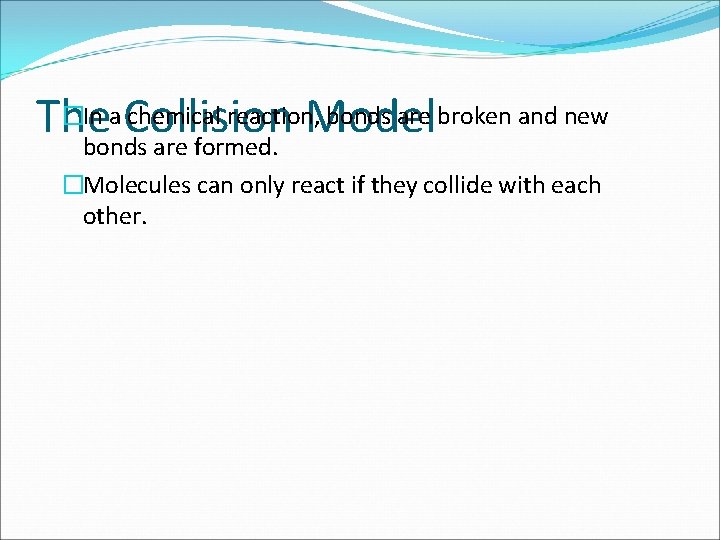
�In a Collision chemical reaction, bonds are broken and new The Model bonds are formed. �Molecules can only react if they collide with each other.
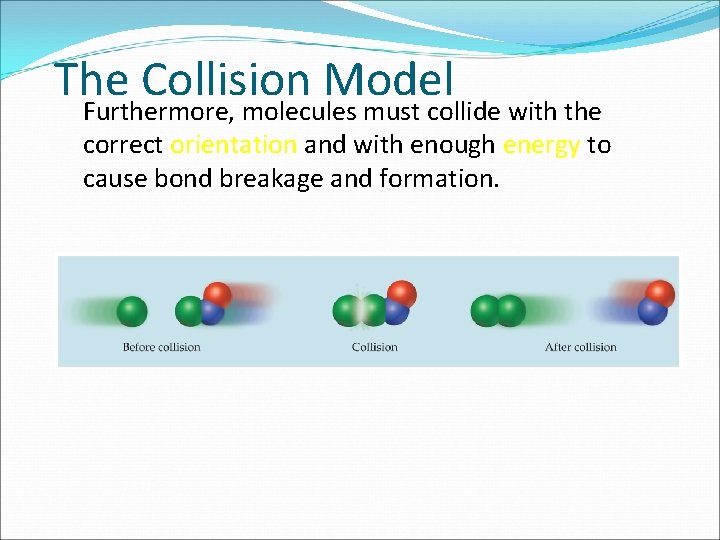
The Collision Model Furthermore, molecules must collide with the correct orientation and with enough energy to cause bond breakage and formation.
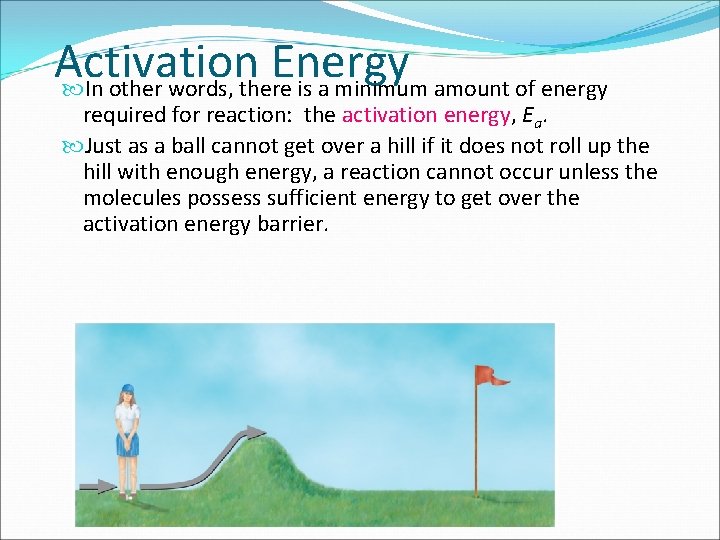
Activation Energy In other words, there is a minimum amount of energy required for reaction: the activation energy, Ea. Just as a ball cannot get over a hill if it does not roll up the hill with enough energy, a reaction cannot occur unless the molecules possess sufficient energy to get over the activation energy barrier.
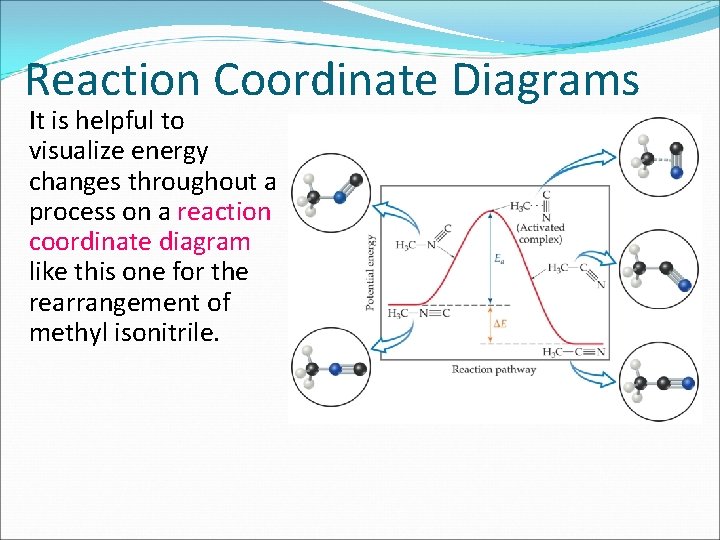
Reaction Coordinate Diagrams It is helpful to visualize energy changes throughout a process on a reaction coordinate diagram like this one for the rearrangement of methyl isonitrile.
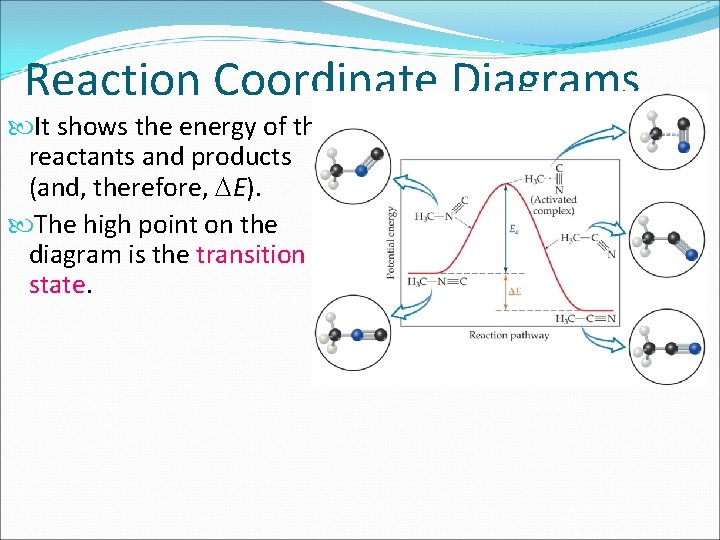
Reaction Coordinate Diagrams It shows the energy of the reactants and products (and, therefore, E). The high point on the diagram is the transition state.
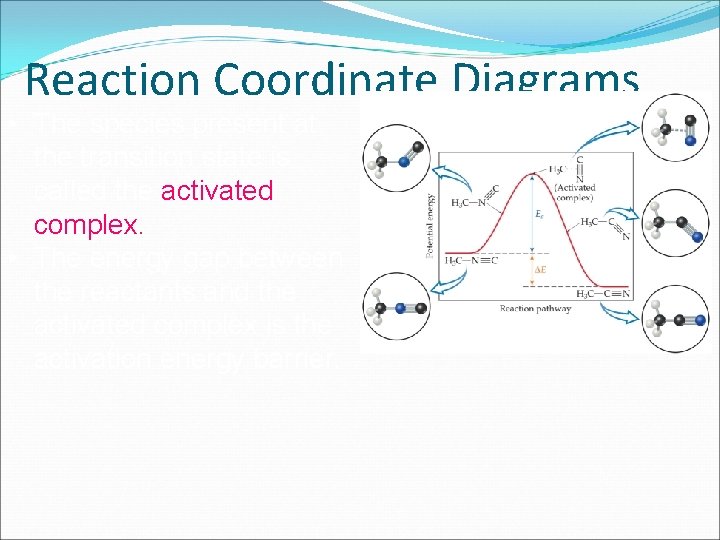
Reaction Coordinate Diagrams • The species present at the transition state is called the activated complex. • The energy gap between the reactants and the activated complex is the activation energy barrier.
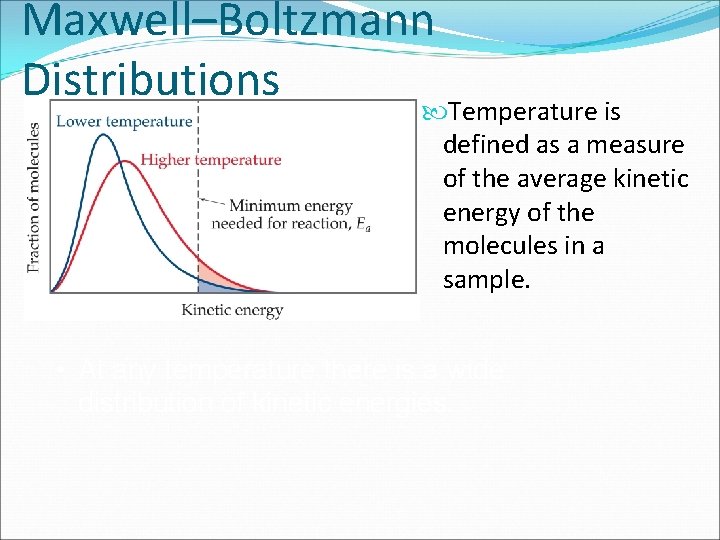
Maxwell–Boltzmann Distributions Temperature is defined as a measure of the average kinetic energy of the molecules in a sample. • At any temperature there is a wide distribution of kinetic energies.
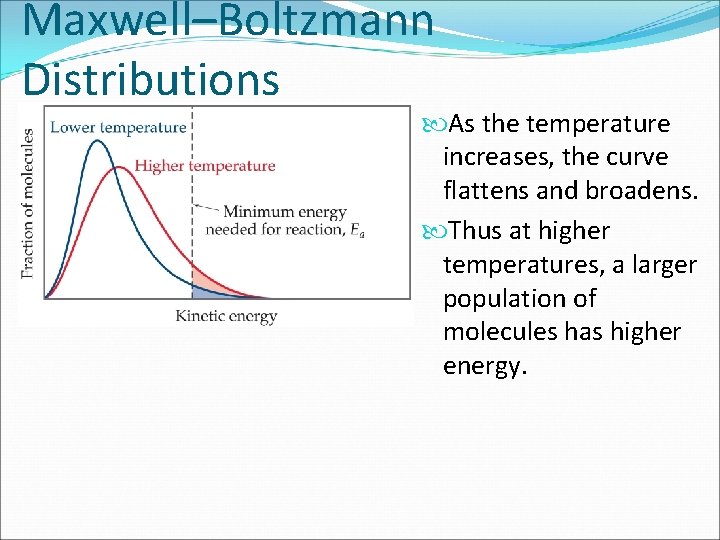
Maxwell–Boltzmann Distributions As the temperature increases, the curve flattens and broadens. Thus at higher temperatures, a larger population of molecules has higher energy.
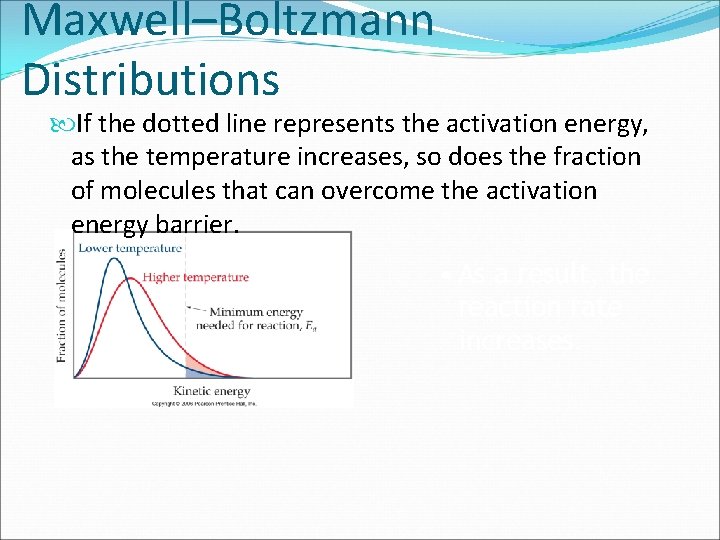
Maxwell–Boltzmann Distributions If the dotted line represents the activation energy, as the temperature increases, so does the fraction of molecules that can overcome the activation energy barrier. • As a result, the reaction rate increases.
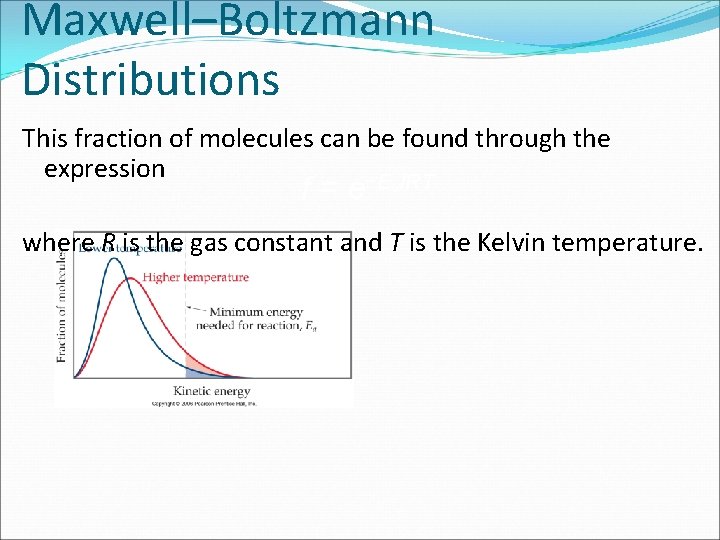
Maxwell–Boltzmann Distributions This fraction of molecules can be found through the expression −E /RT f=e a where R is the gas constant and T is the Kelvin temperature.
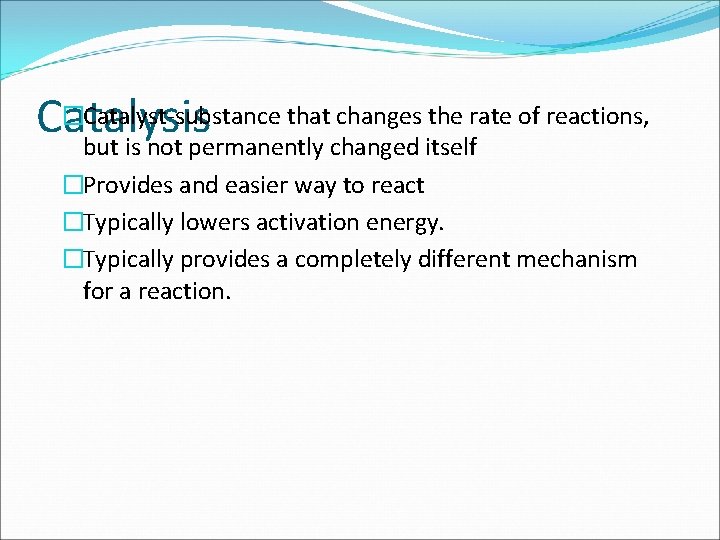
�Catalyst-substance that changes the rate of reactions, Catalysis but is not permanently changed itself �Provides and easier way to react �Typically lowers activation energy. �Typically provides a completely different mechanism for a reaction.
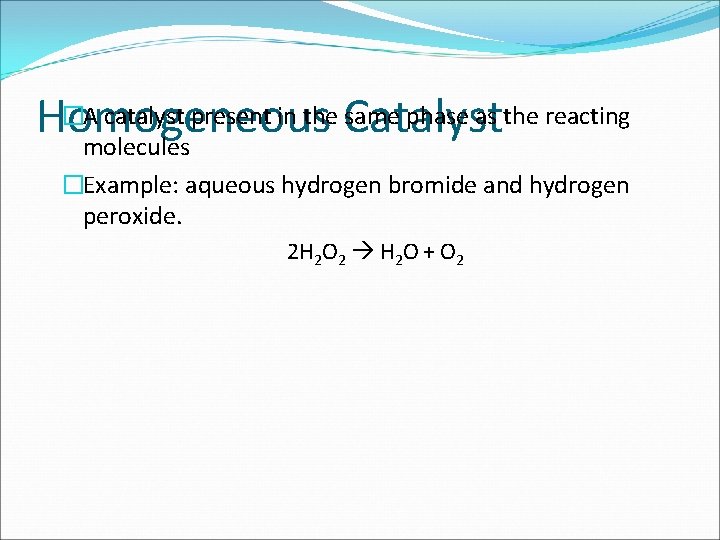
�A catalyst present in the Catalyst same phase as the reacting Homogeneous molecules �Example: aqueous hydrogen bromide and hydrogen peroxide. 2 H 2 O 2 H 2 O + O 2
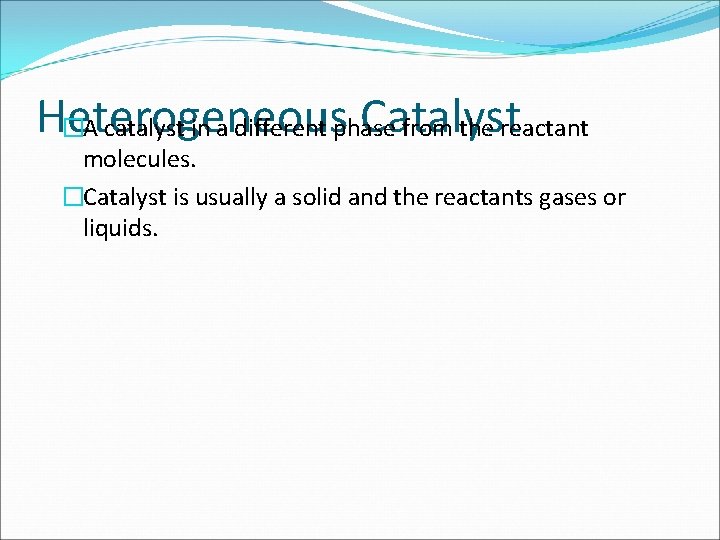
Heterogeneous Catalyst �A catalyst in a different phase from the reactant molecules. �Catalyst is usually a solid and the reactants gases or liquids.
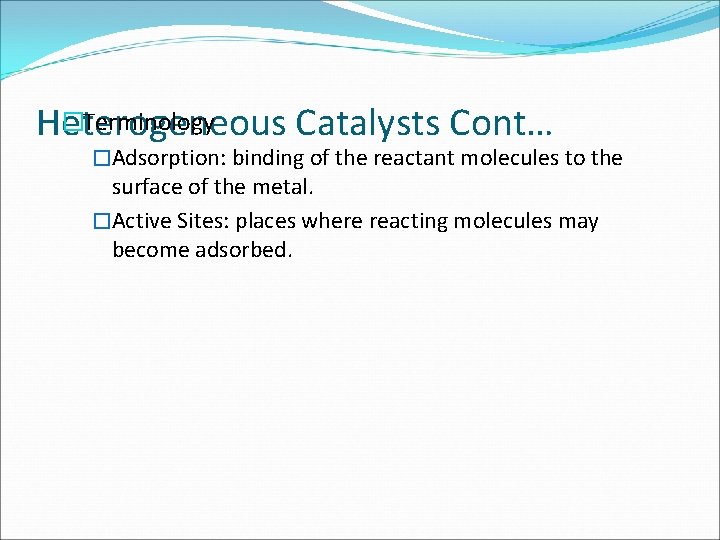
�Terminology Heterogeneous Catalysts Cont… �Adsorption: binding of the reactant molecules to the surface of the metal. �Active Sites: places where reacting molecules may become adsorbed.
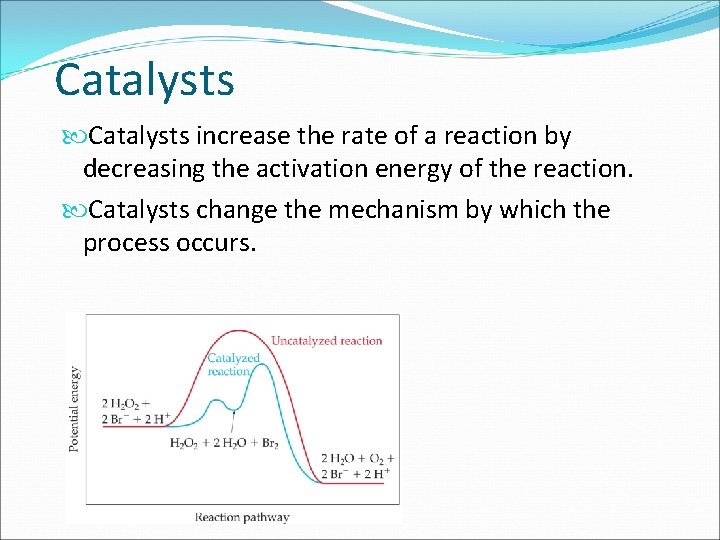
Catalysts increase the rate of a reaction by decreasing the activation energy of the reaction. Catalysts change the mechanism by which the process occurs.
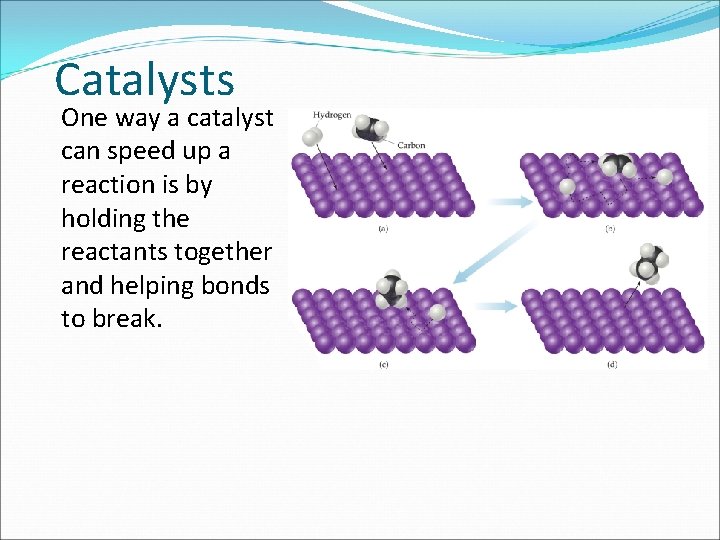
Catalysts One way a catalyst can speed up a reaction is by holding the reactants together and helping bonds to break.
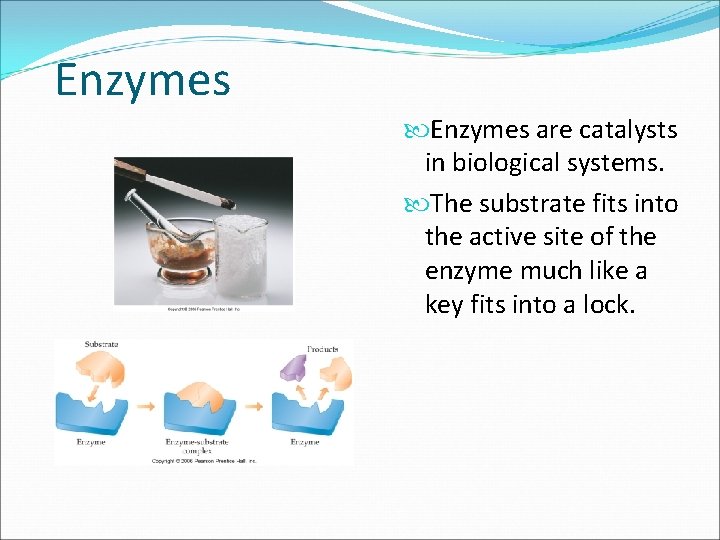
Enzymes are catalysts in biological systems. The substrate fits into the active site of the enzyme much like a key fits into a lock.
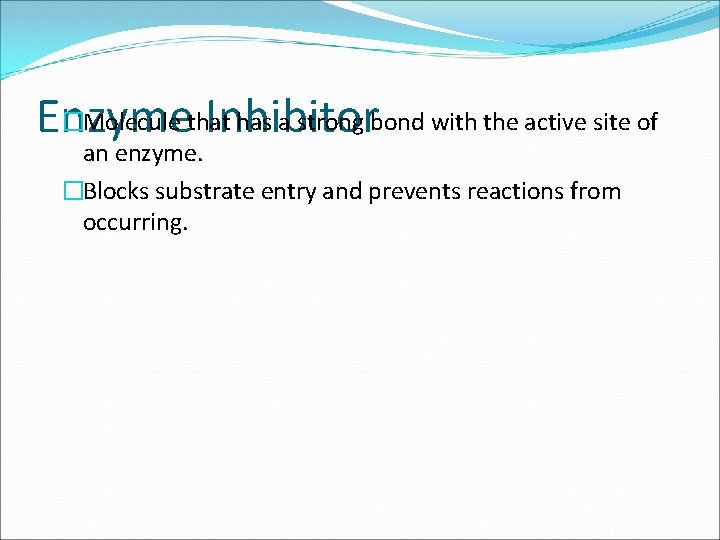
�Molecule that has a strong bond with the active site of Enzyme Inhibitor an enzyme. �Blocks substrate entry and prevents reactions from occurring.
Chemical reactions grade 11
Applications of chemical kinetics
Half life kinetics
Steady state kinetics
Chemical kinetics definition
Definition of chemical kinetics in chemistry
Chemical kinetics experiment
Now i see it now you don't
Amer rasheed
Chapter 2 jesus christ true god and true man
True or false: chemical and physical changes alter matter.
Lesson 3 physical and chemical changes answers
Rate of growth
Kinetics of particles newton's second law
Enzyme kinetics
Kinetic of rigid body
Kinetics and equilibrium
Kcat
Octet kinetics
Collision theory of kinetics
Kinetics is the branch of:
Kinetics of a particle: impulse and momentum
Abbas et al
Kinetics of a particle force and acceleration
Prostagalndins
Planar kinetics of a rigid body work and energy
Km in enzyme kinetics
Kinetics flotation chemicals
Cell kinetics and fermenter design
Dynafit software
Kinematic of rigid body
Ap chem kinetics practice problems
Difference between zero and first order kinetics
Plane movement
Kinetics reaction
Michaelis menten graph inhibitors
Planar kinetics of a rigid body: force and acceleration
Vampnets for deep learning of molecular kinetics
Data kinetics ltd
Kinetics of crystal violet fading
First order drug elimination
Chemical reactions section 1 chemical changes
Section 2 classifying chemical reactions
Chapter 7 chemical formulas and chemical compounds test
Chapter 7 review chemical formulas and chemical compounds
Section 2 classifying chemical reactions worksheet answers
Chapter 18 chemical reactions balancing chemical equations
Lets practice
Paper due now
Present indefinite tense
She is reading a newspaper
Mobile and pervasive computing
Kids read now
Ah faustus now hast thou
Waller ffa now
Cern service now
I can't get no satisfaction
This is amazing grace hillsong
Now all has been heard here is the conclusion of the matter
I am originally from the south but now i live in the north
What is a do now activity
Pentecost
Fpl solar now
Holidays then and now
Real noah's ark
Now and then board
Enunciation practice
Computer graphics models are now commonly used for making
Because the tomb is empty
Geography now canada
How is janie able to tolerate her relationship
Now fill in the blanks
Lesson 7 act 3 scenes 1-3
Hooray cheered gilda. does that mean we can stay?
Bce to ce timeline
Present past future continuous tense examples
Eye can see clearly now
"reachnow.com"
I had ____ fish and ____ chips for dinner
Now lifestyle autoresponder
My body changes
Harry poter test
Confirm your email now and get 5 minutes as a gift!
Bond angle degrees
Starteam api
I am eating now
Vlt gang
Reggie stephey
Social studies do now questions
John purdy folding chair
Troy now
Not now
My father said i could use his car
Announcement flag ceremony
Infohub uwe
Hear him now passive voice
From now
The power process be here now
Now answer these questions
Uwa service desk
Aim do now
Abb.service-now/myis
Systems applications products
Anthony swindells now
Everybody ready to start now
Now listen and repeat
I write a letter now past continuous tense
Is now a time order word
He invented bean bags and wooden dumbells
Bc to now timeline
Ffa historian symbol
Myinprsretirement.org register now
What is sociology section 2
Mullet fingers now
Nursing now today's issues tomorrow's trends
And think this heart all evil shed away
Jac colon revelation now
Complete the following table element
Philosophy is odious and obscure
Let's begin our discussion
He probably loves (you) now
Ticket giver kenning
Tuntutan pedagogis pkn di sd
Waste management (now wm) - wichita hauling
Hide me now tagalog lyrics
Naver.com news
Where is captain nielsen from
Service now cern
Jerusalem then and now
Which way now
Act out the dialogue
Nnn now
Now write a slogan using bandwagon techniques.
The film is very interesting
Sturmabteilung
Shh-hh he'll be quiet now maybe analysis
Martyn lowery
Agriculture now and then
Modal verbs structure
I will be still know you are god
Seaside holidays then and now
Now dream
Stop it now modules
Shows only my teeth like a snake bare fangs
Smsu eservices login
Dexieume
Answer the question write your answer on your notebook
Advantages of icon based authoring tool
Calorimeter
The darkness drops again but now i know
My son _____ be home by now. where can he be?
Formation of neural networks ib psychology
Remains annotations
How often do you play it
Ant and seb now
If + past simple
Windows connect now
Now upon the first day of the week
What is the ending of goldilocks and the three bears
Then and now grammar
Levinson life stages
Servicenow problem management
Hippa secure now
Turkan soray now
τίνινγκ τζόι
New york state mesonet
Released now fullblown multimedia authoring system
Teori here and now
"hi steve. what are you doing here?"
Edmund from narnia now
Semi realistic mannequins
Hilly bourn
What quite unmanned in folly