PHAR 2811 Dales lecture 7 The Transcriptome Synopsis
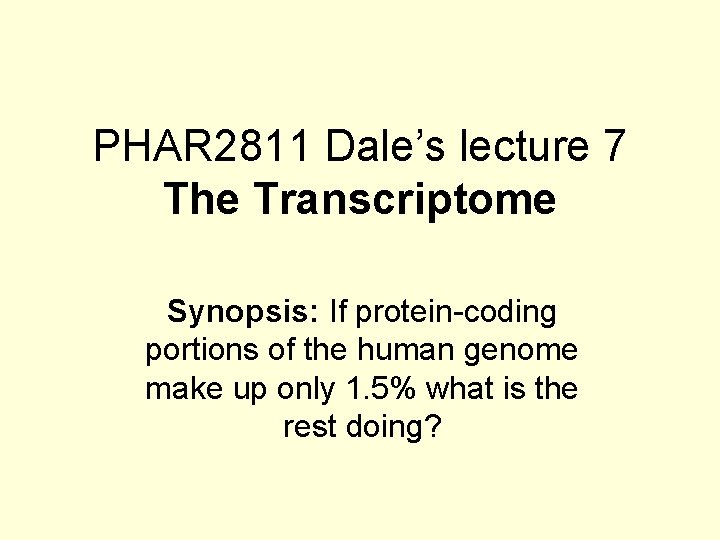
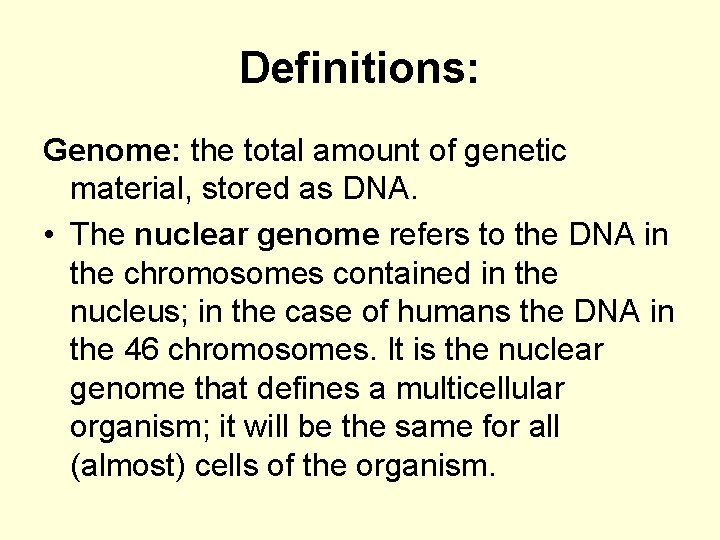
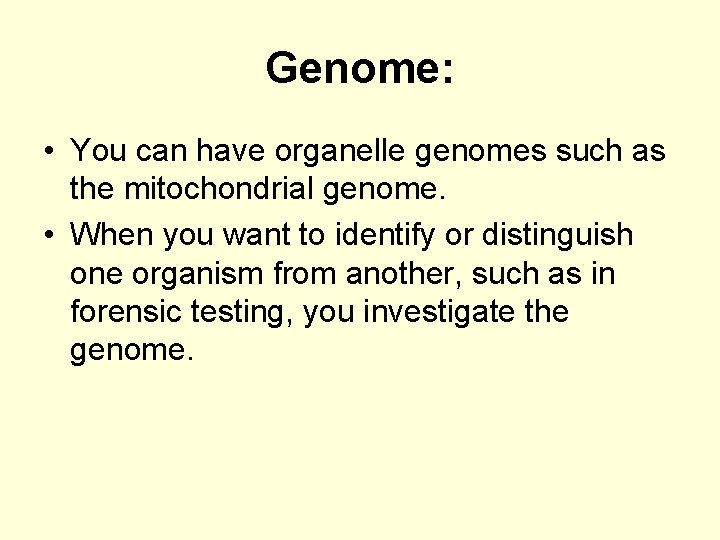
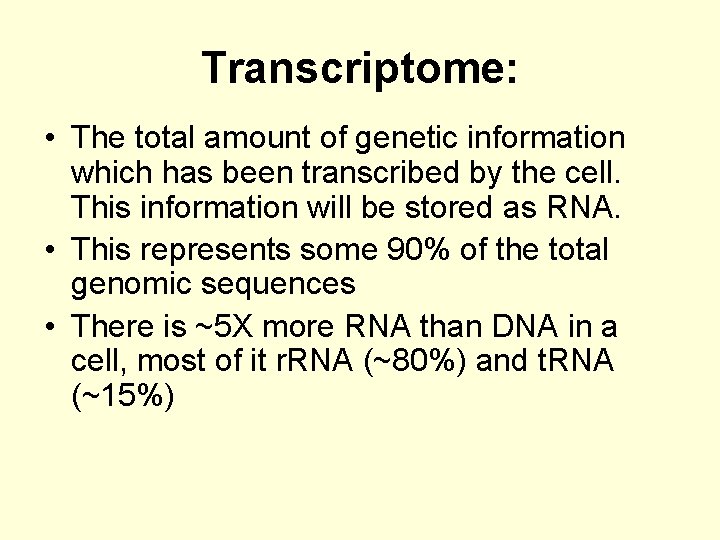
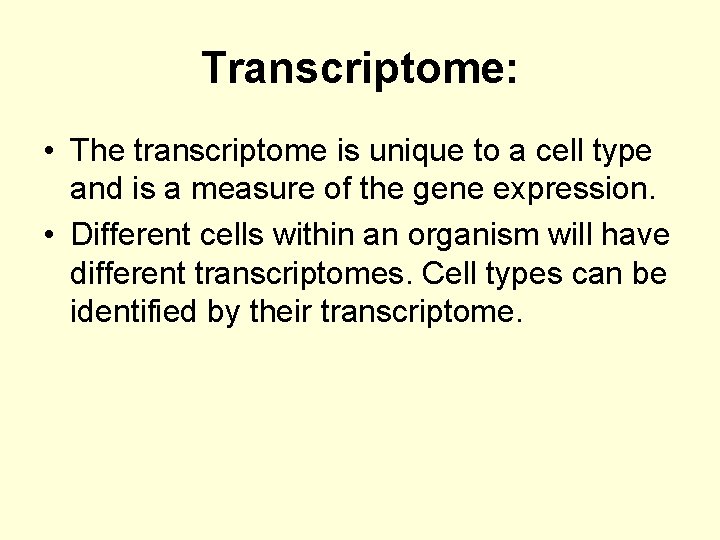
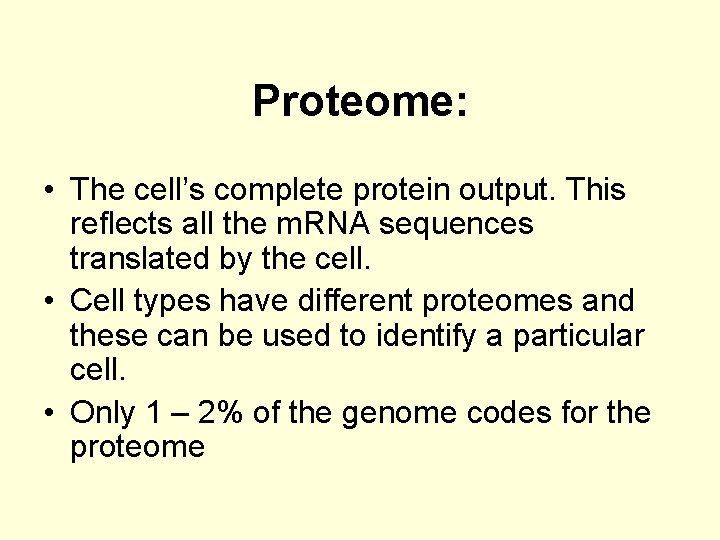
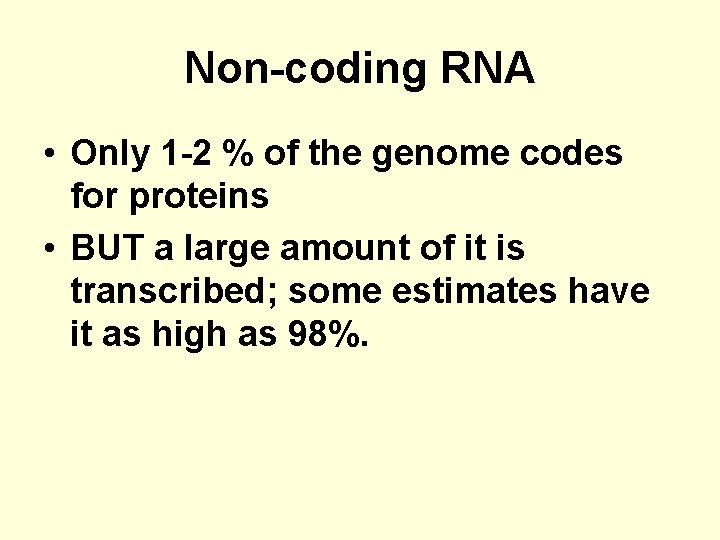
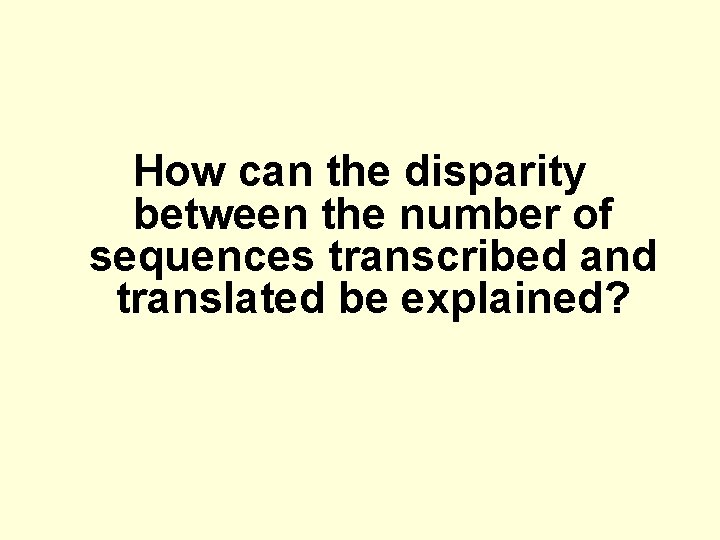
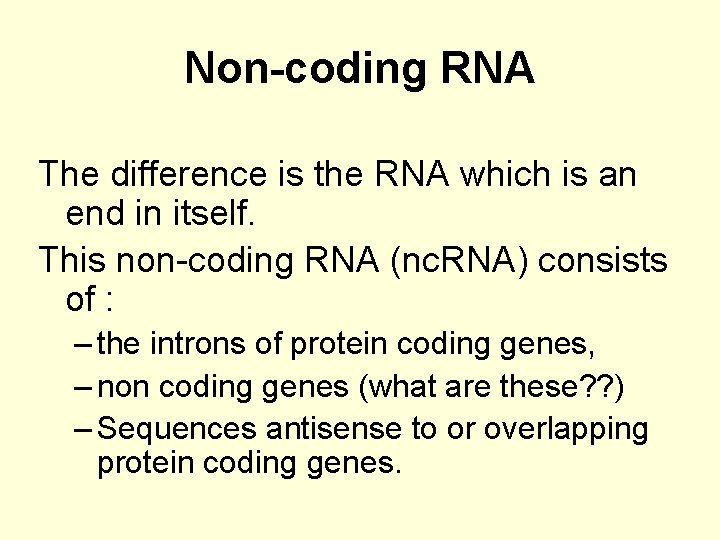
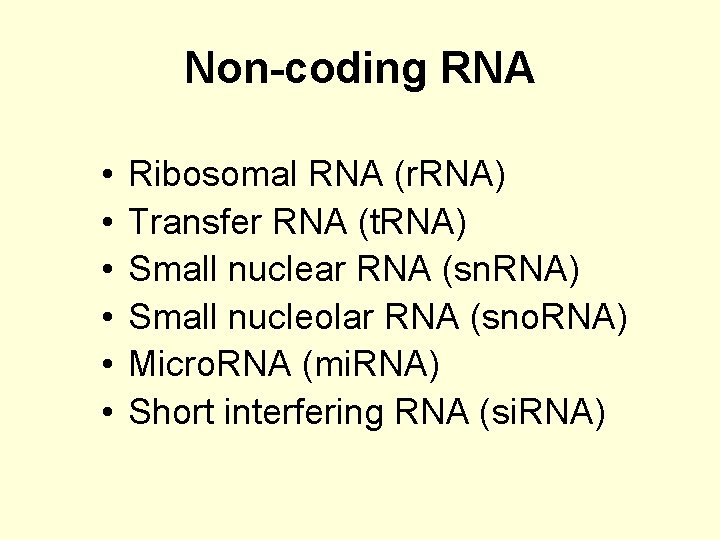
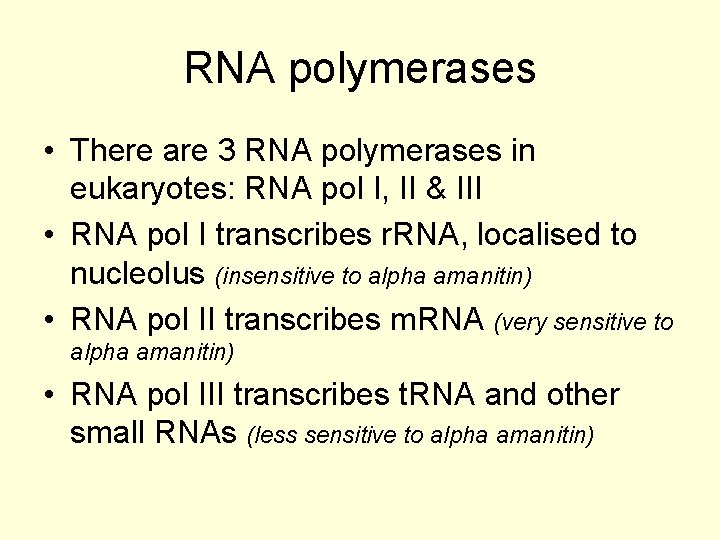
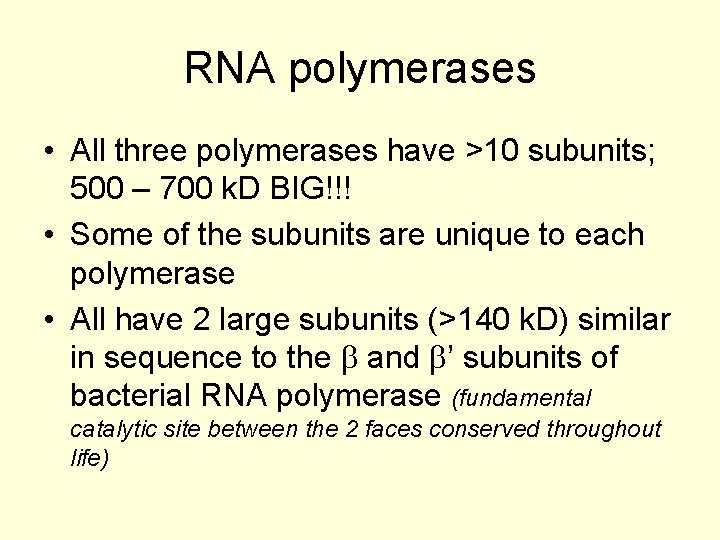
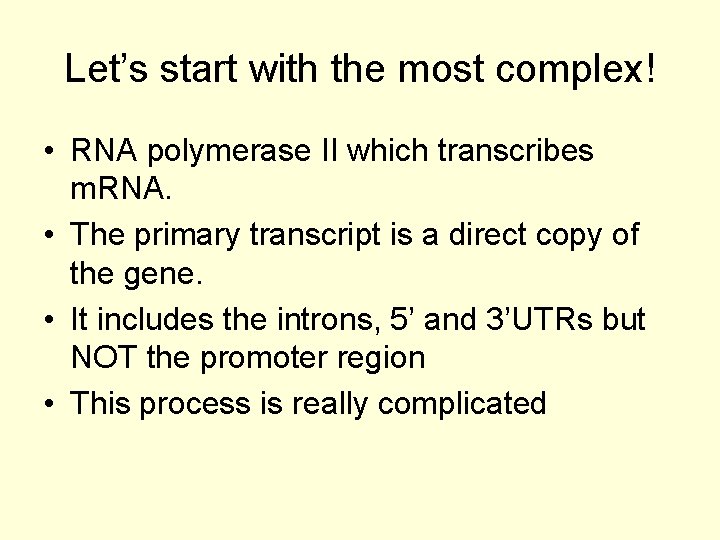
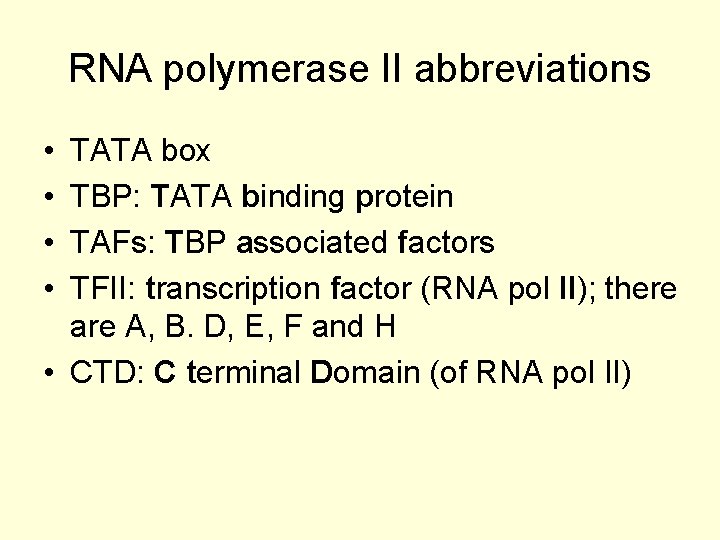
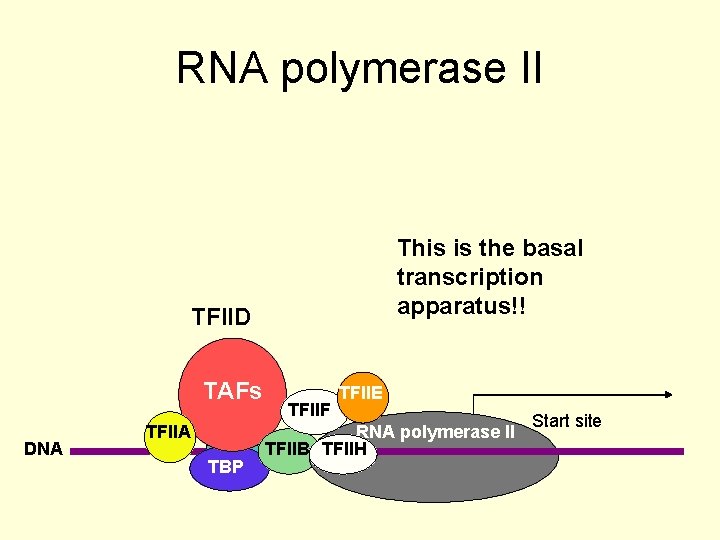
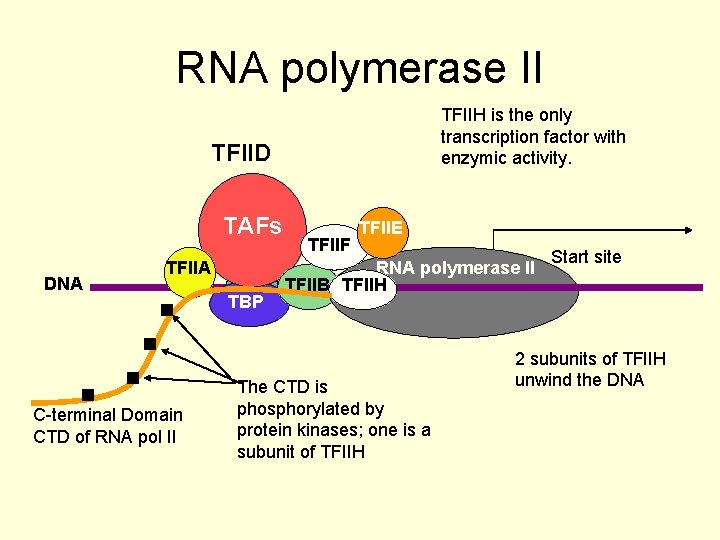
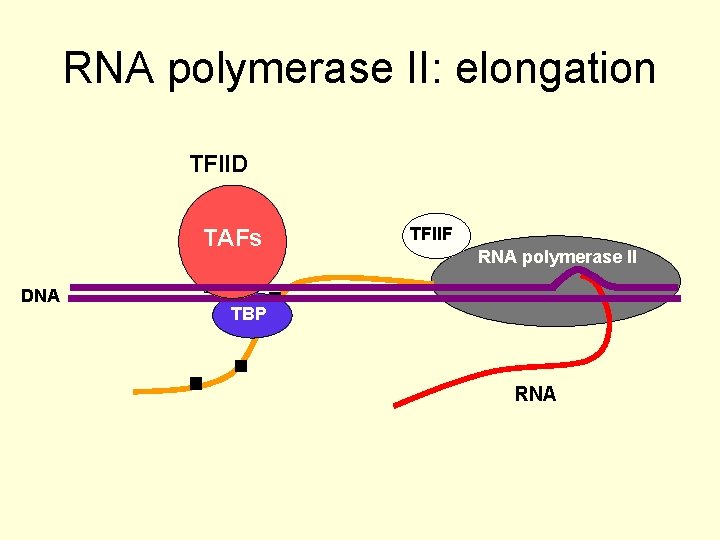
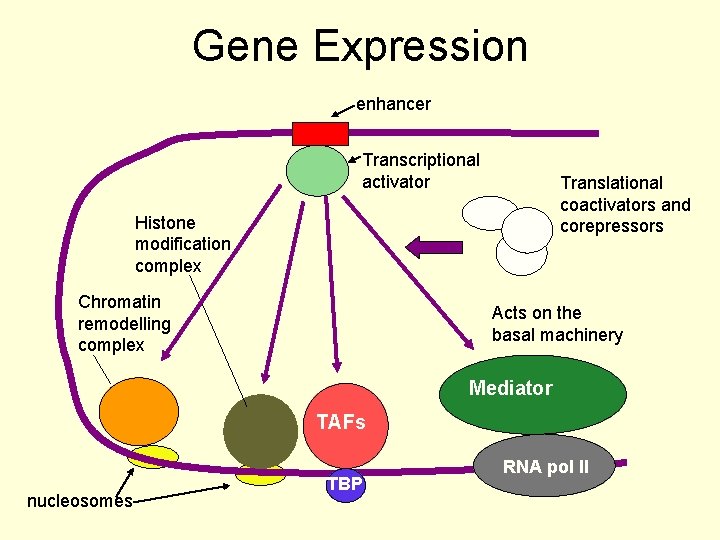
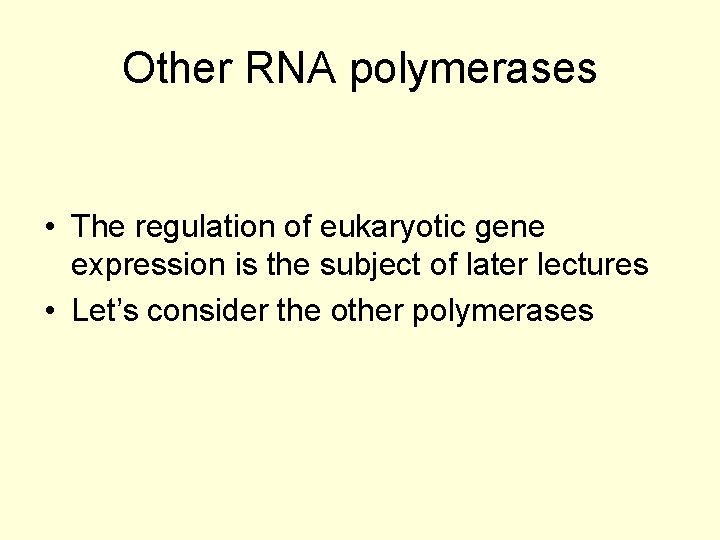
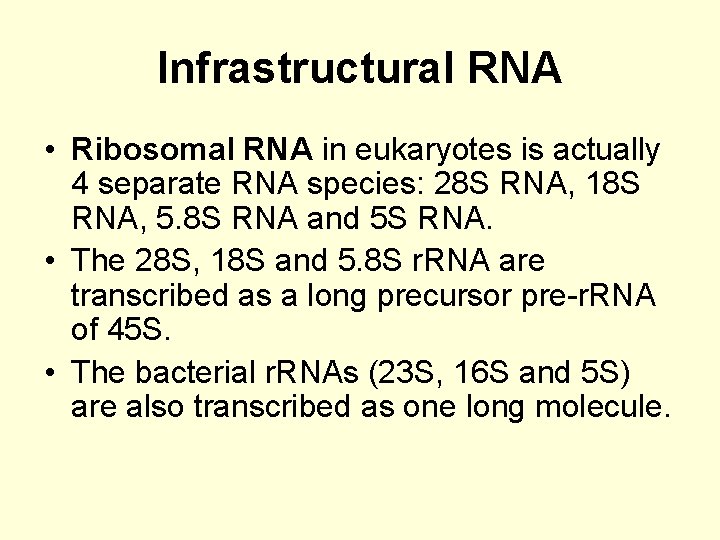
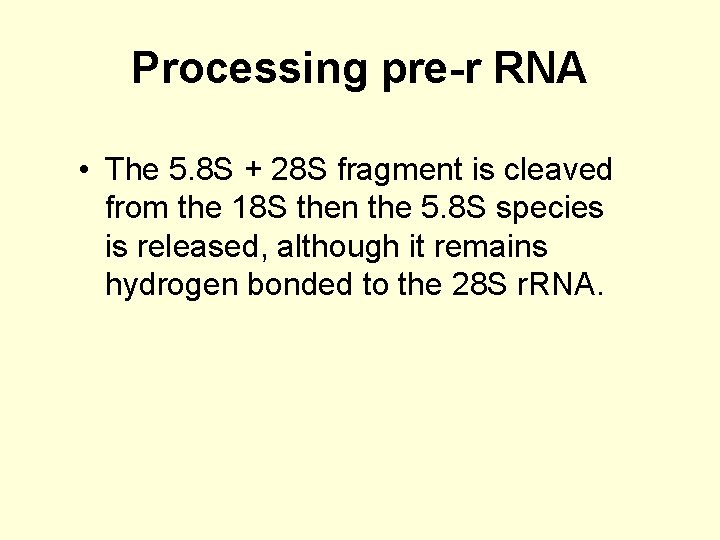
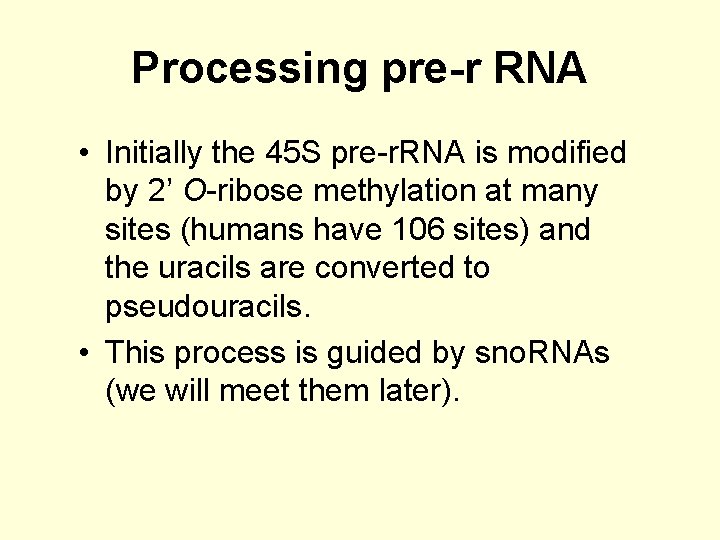
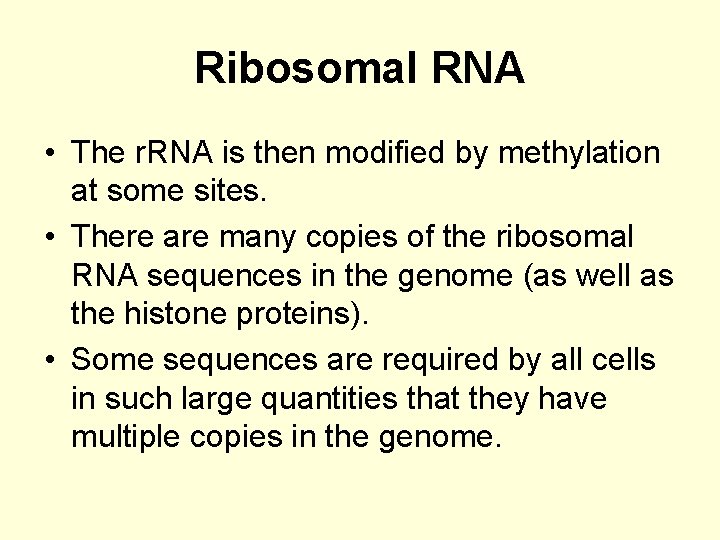
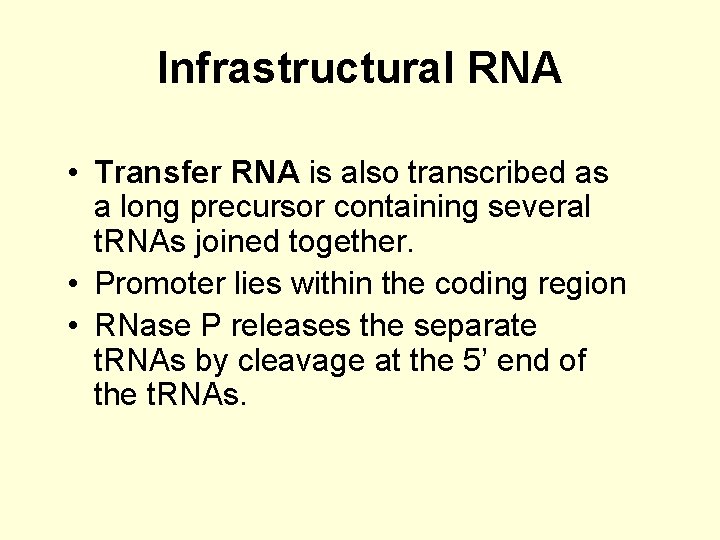
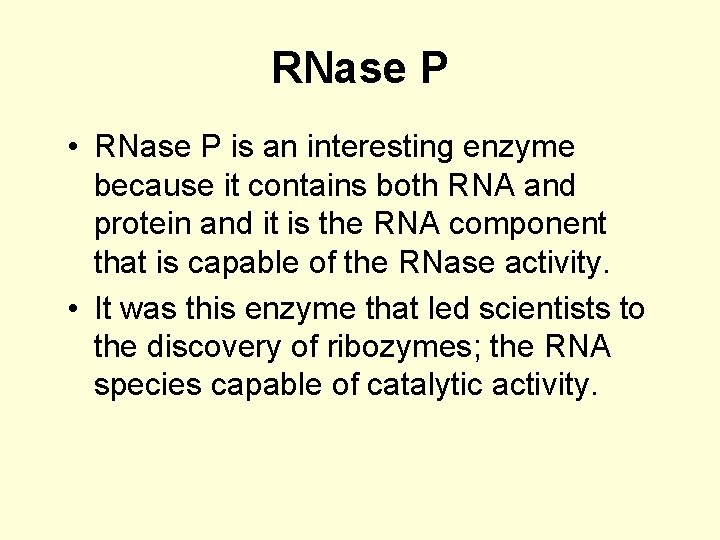
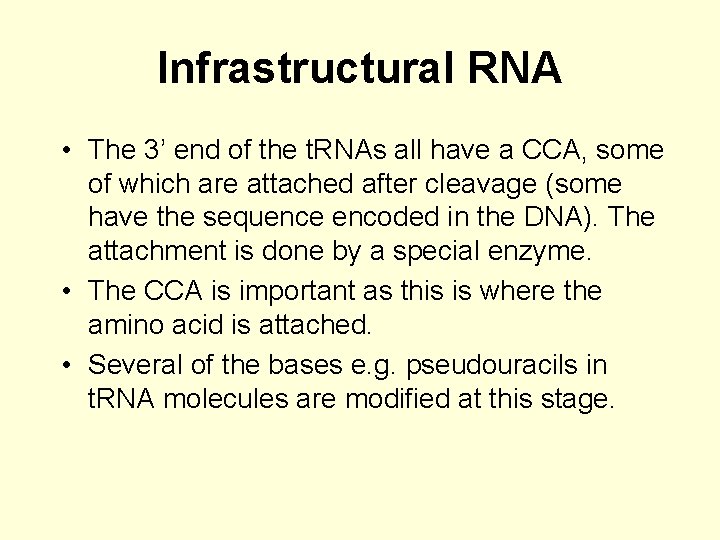
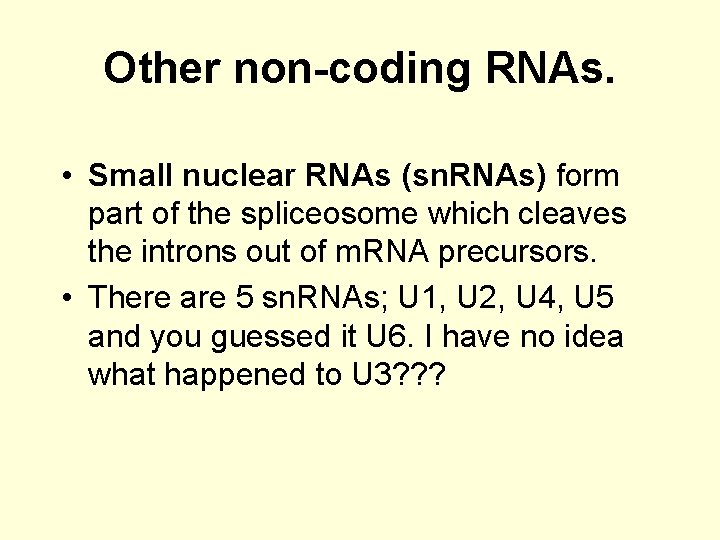
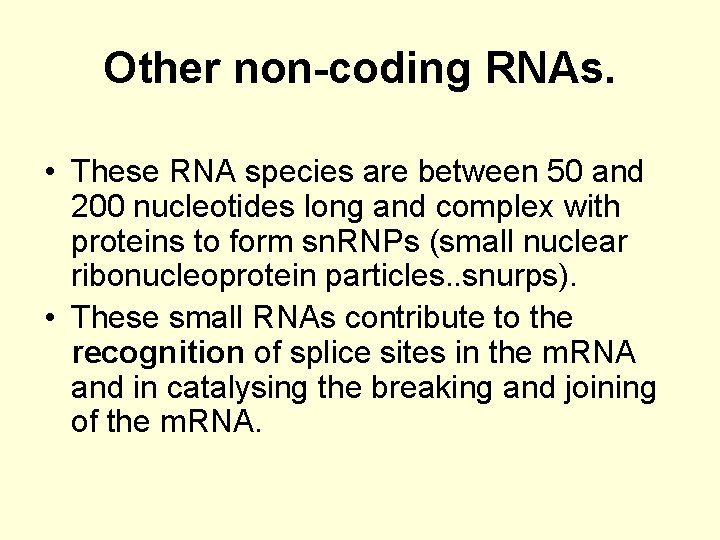
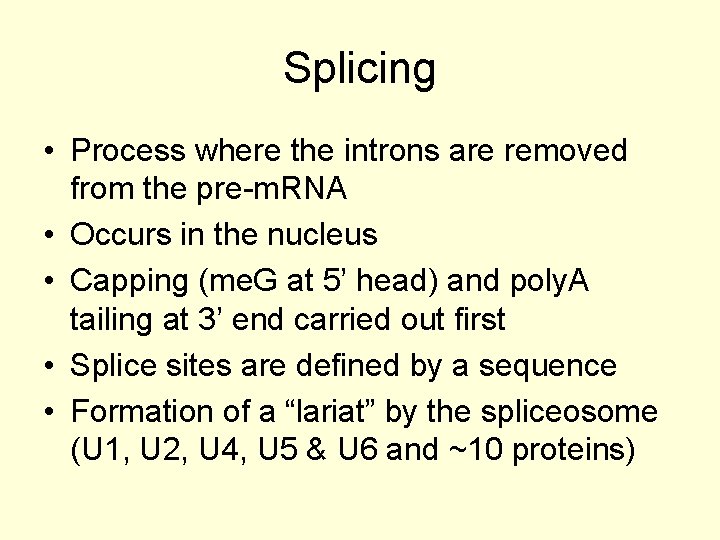
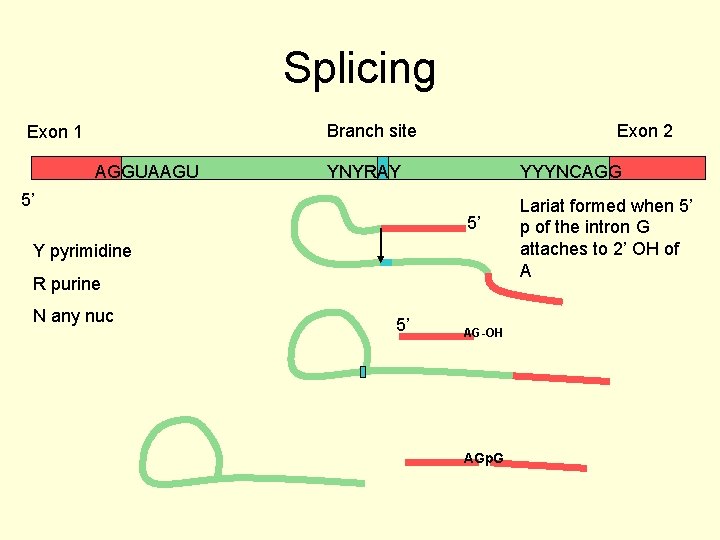
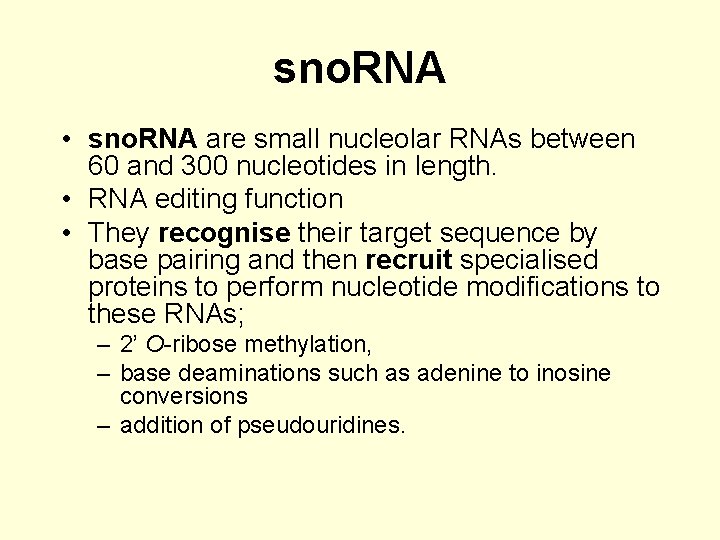
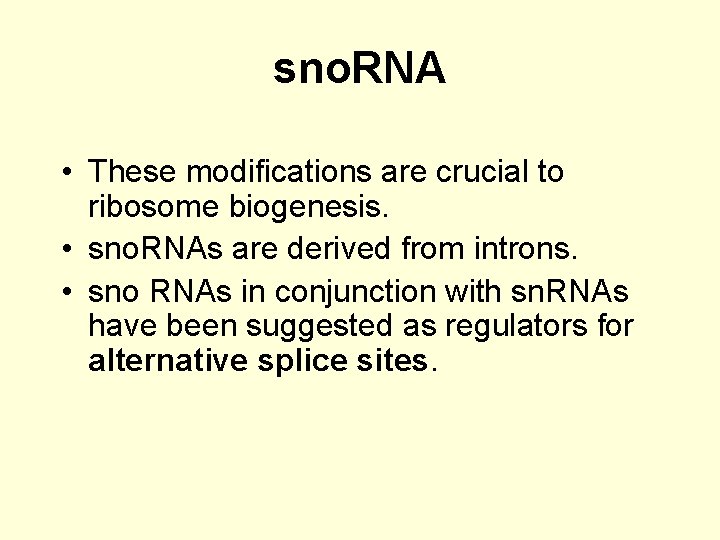
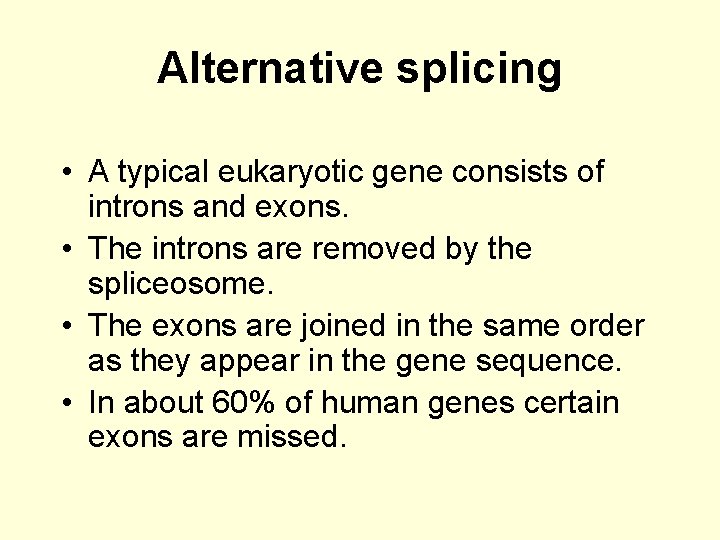
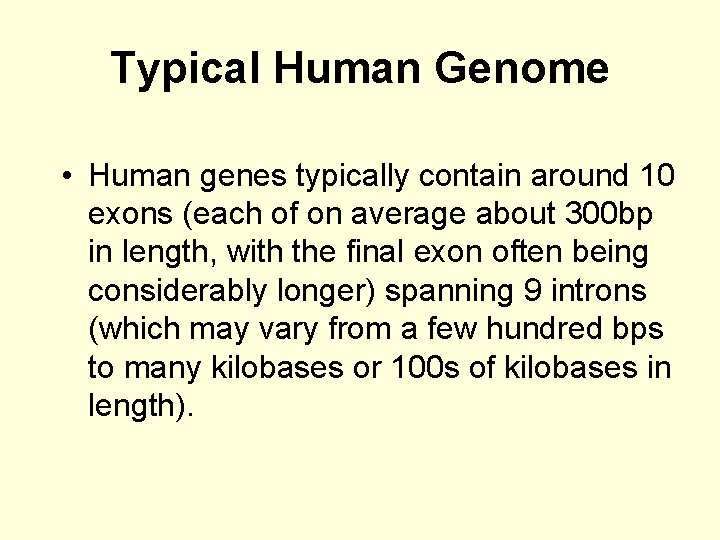
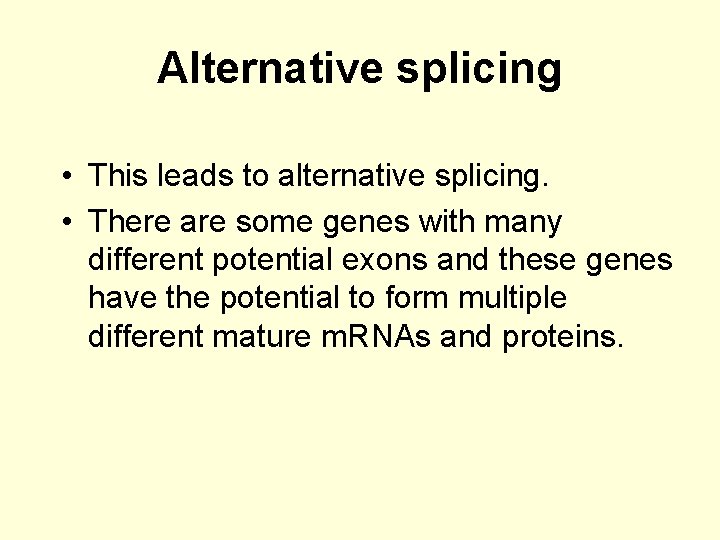
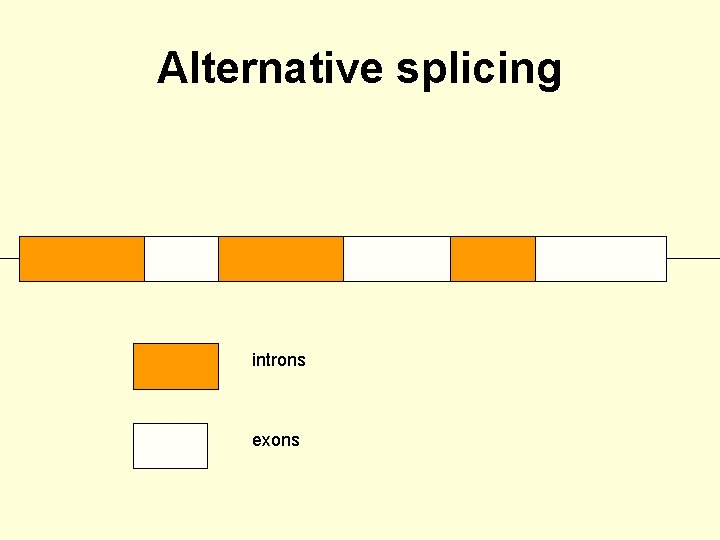
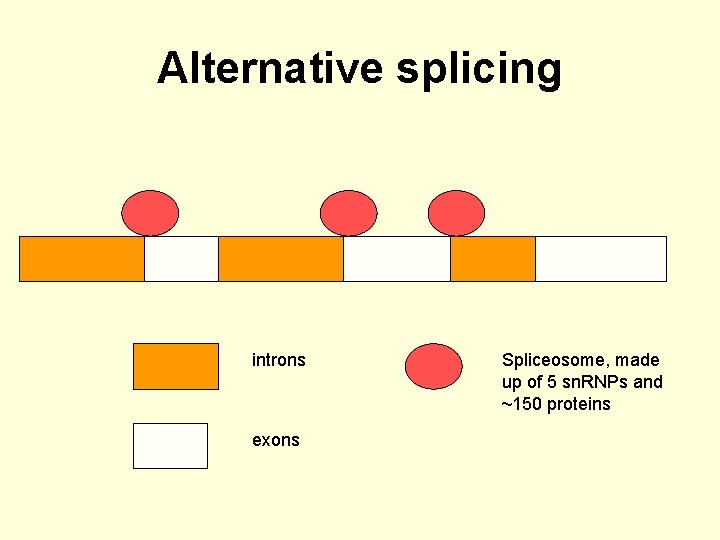
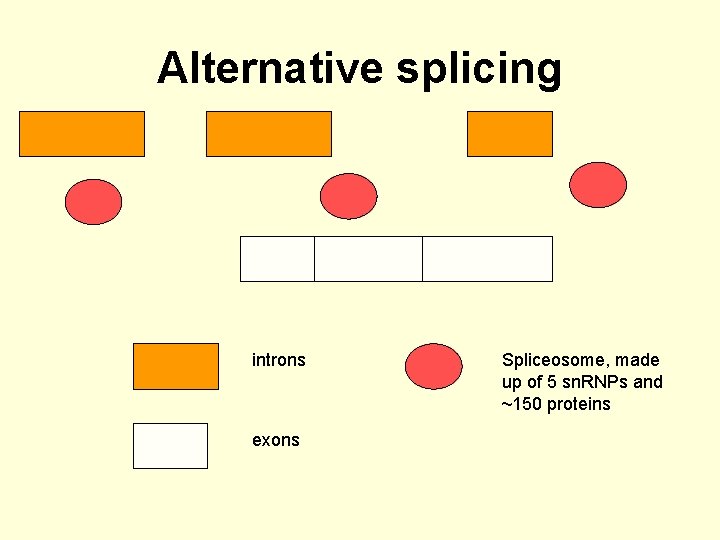
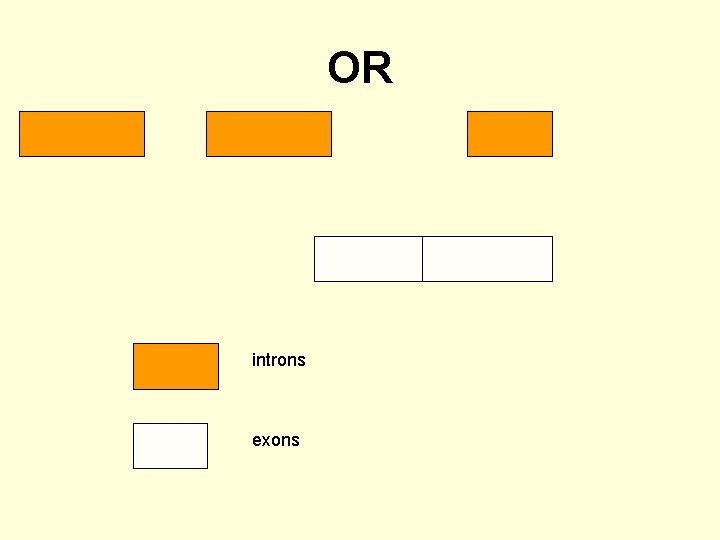
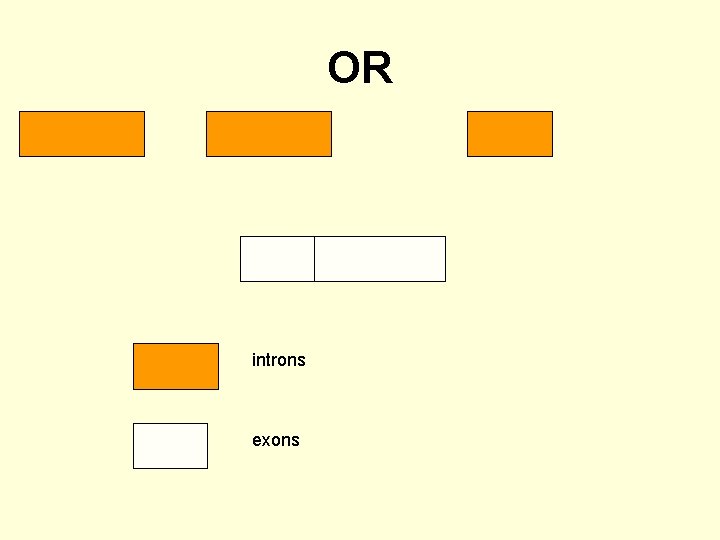
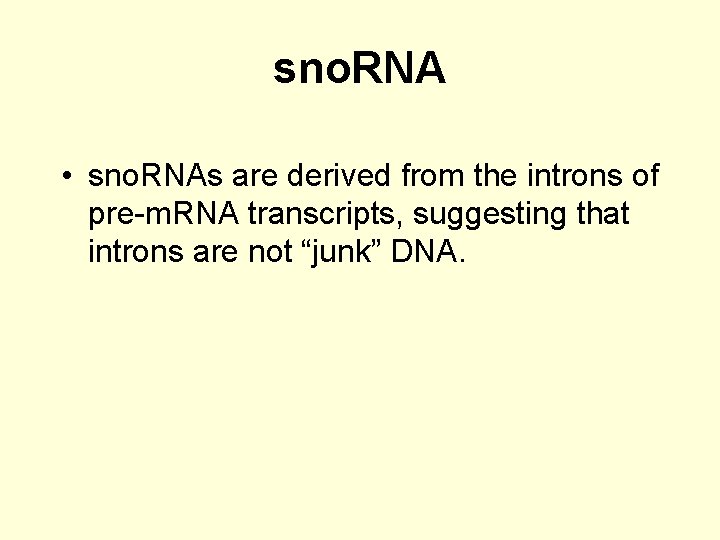
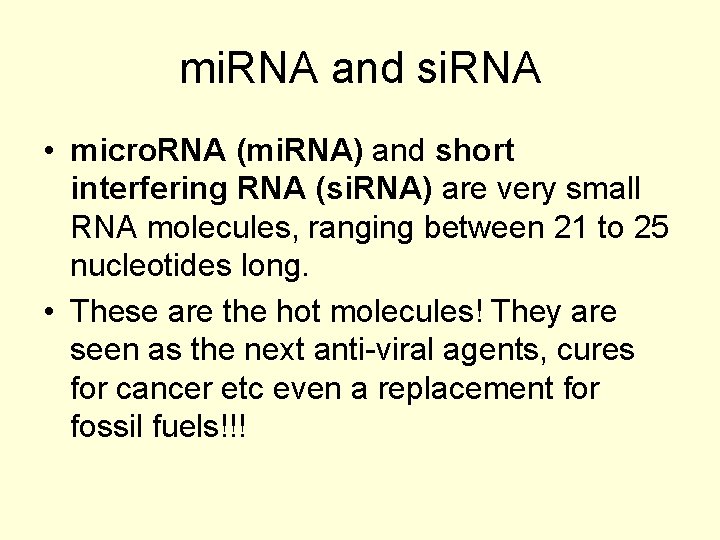
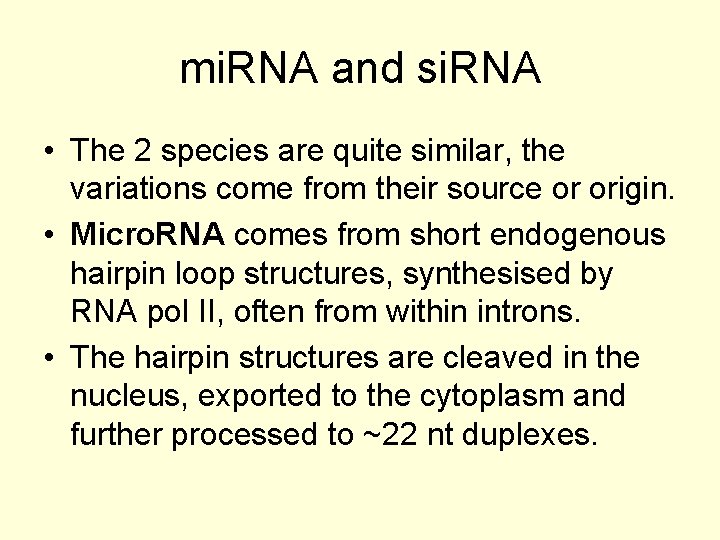
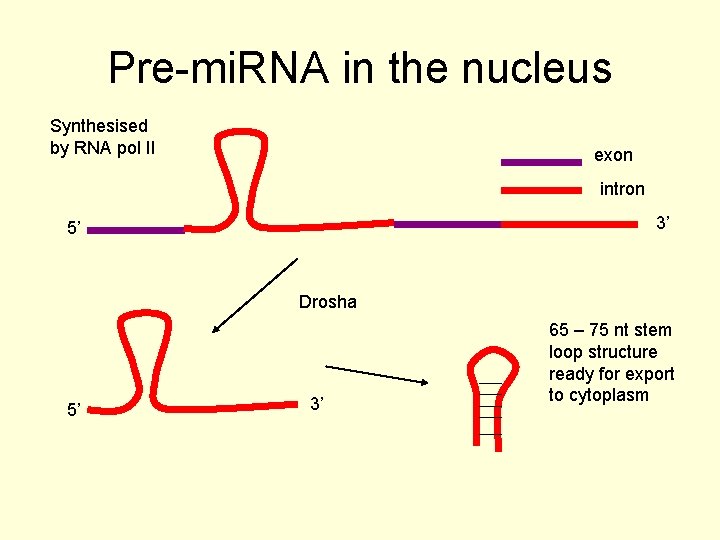
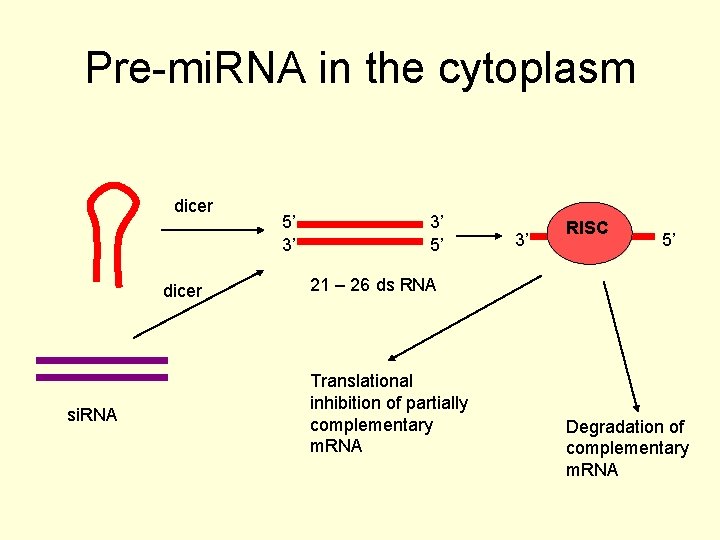
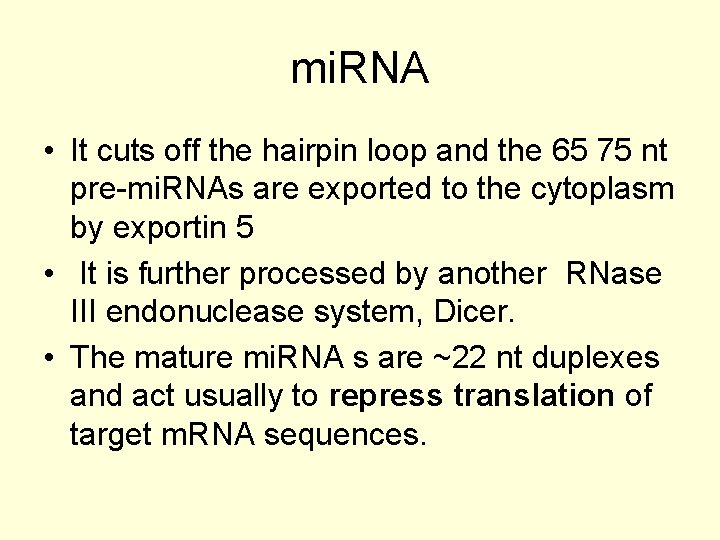
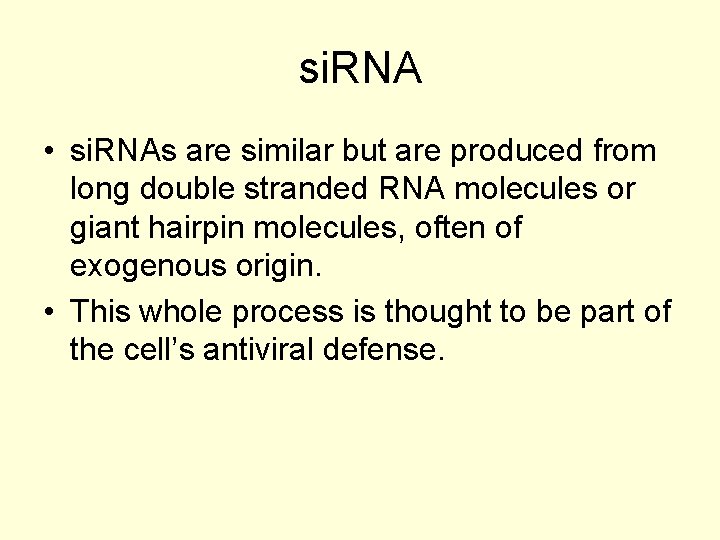
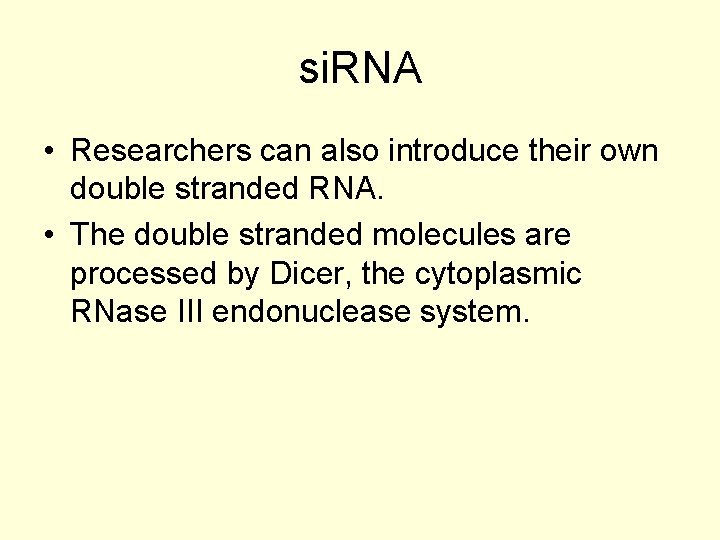
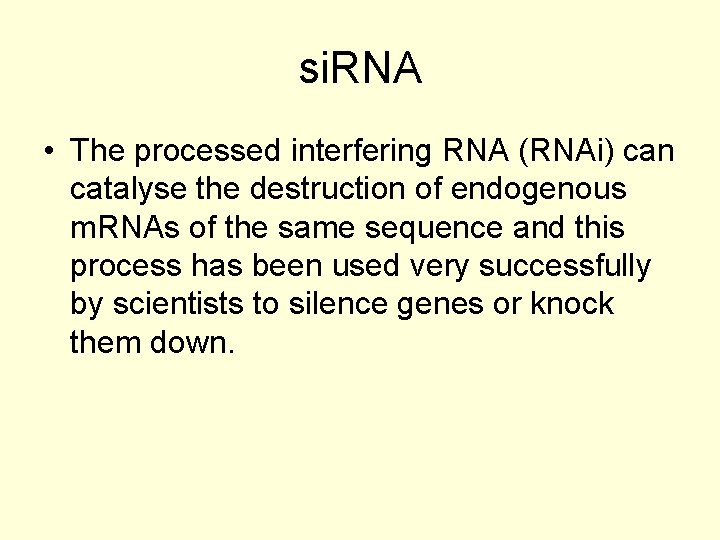
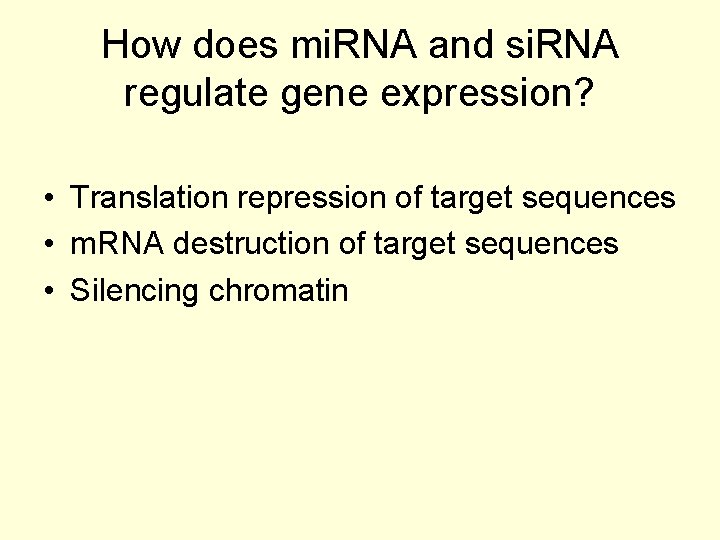
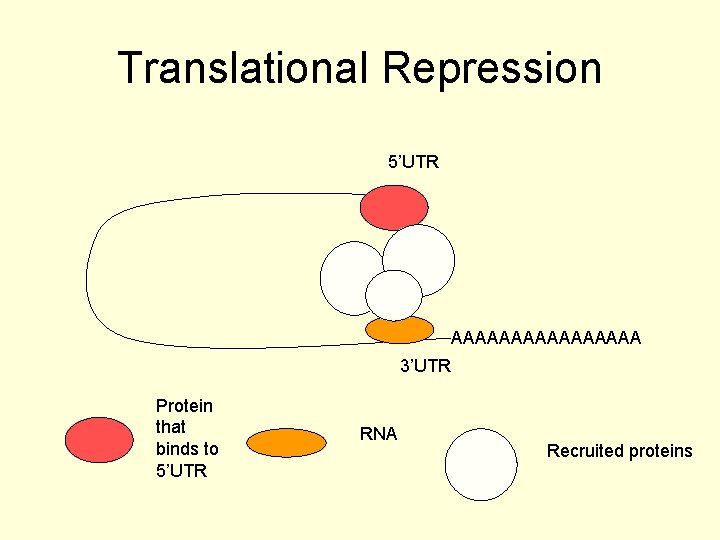
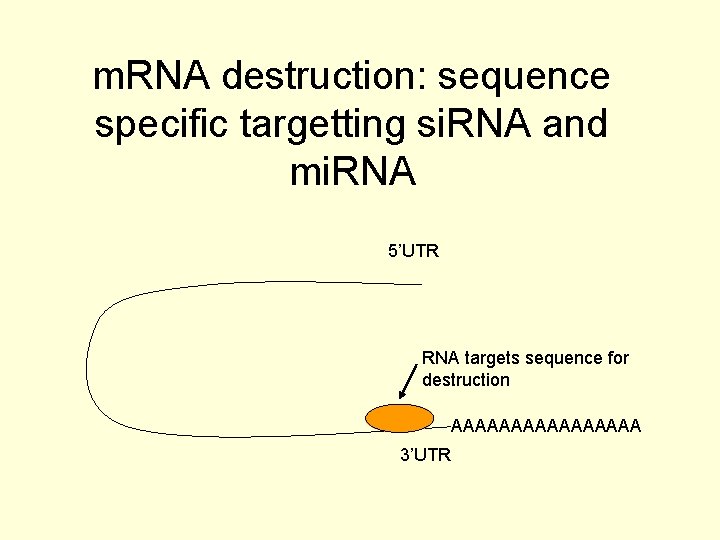
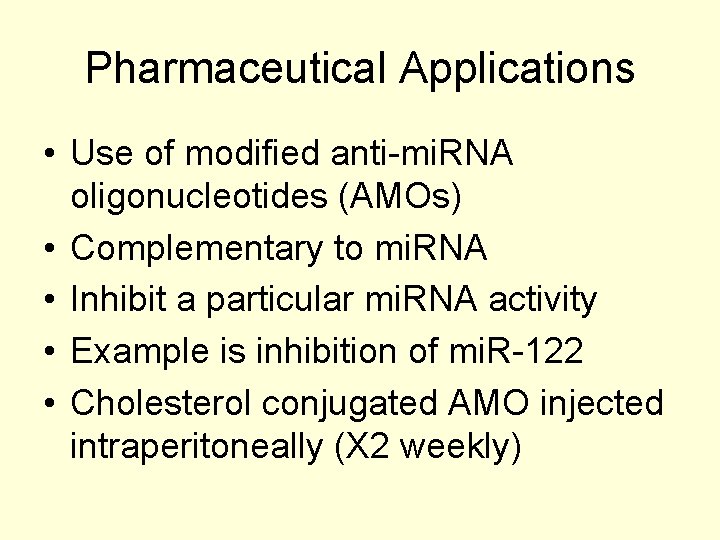
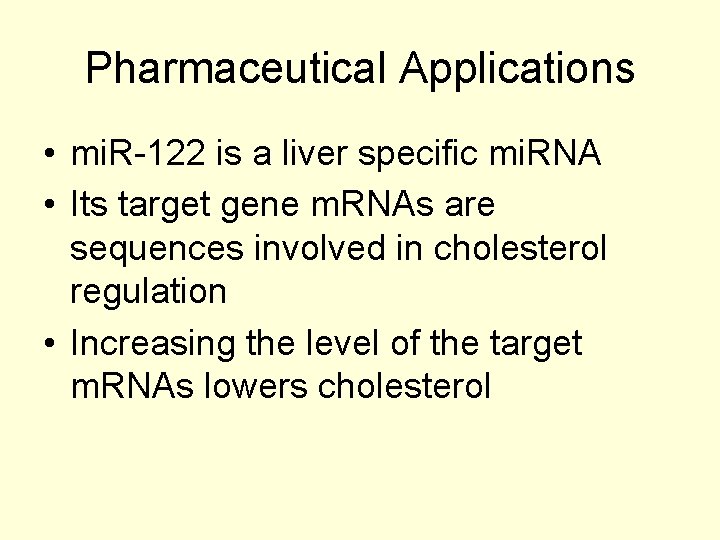
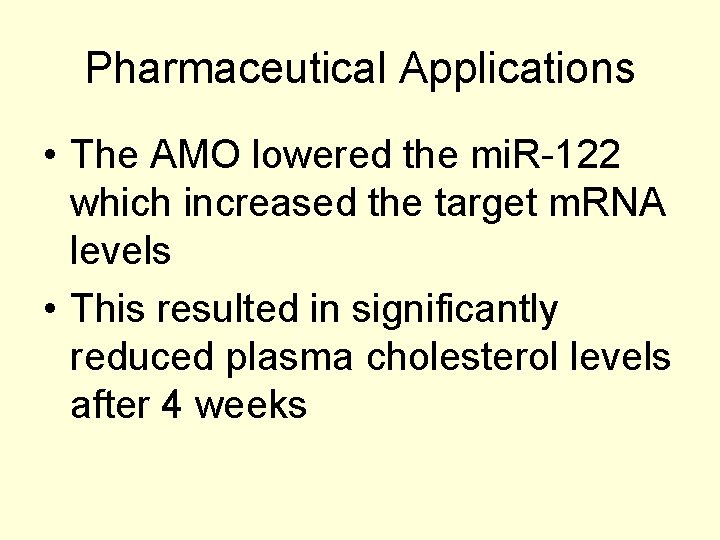
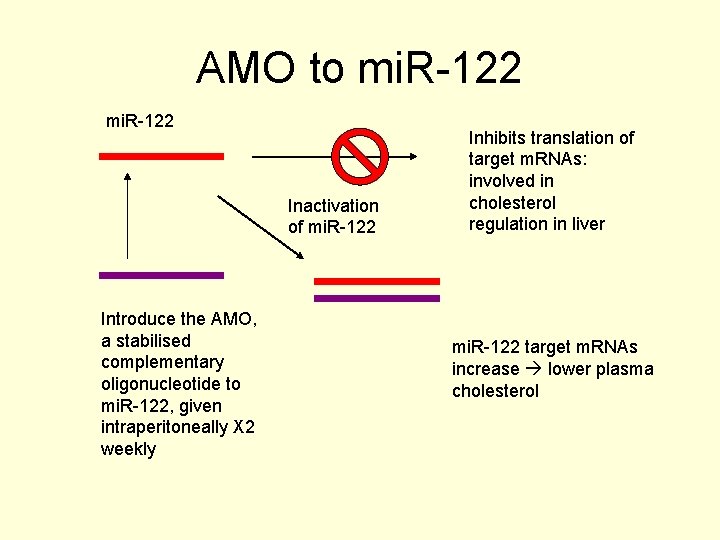
- Slides: 56
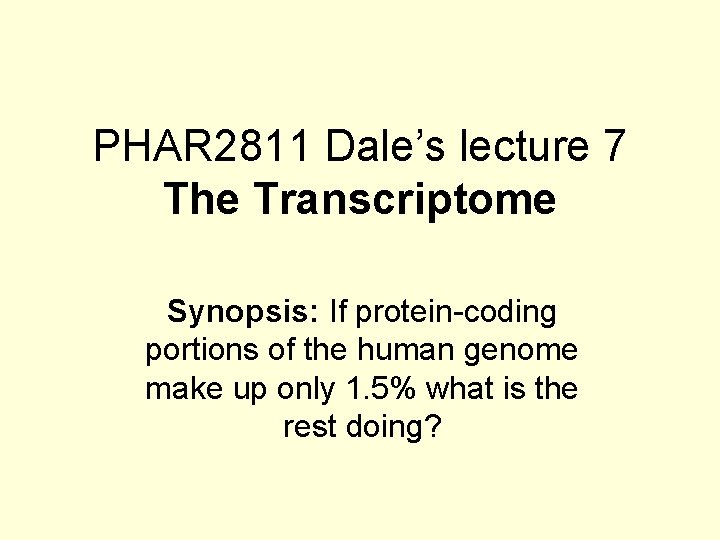
PHAR 2811 Dale’s lecture 7 The Transcriptome Synopsis: If protein-coding portions of the human genome make up only 1. 5% what is the rest doing?
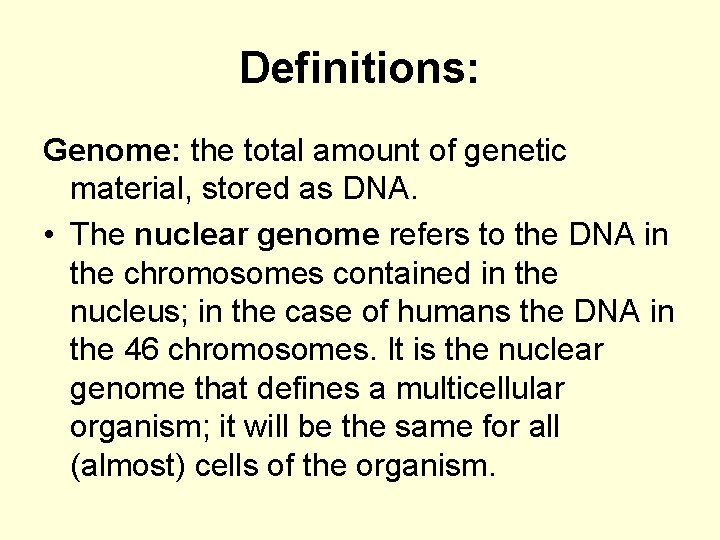
Definitions: Genome: the total amount of genetic material, stored as DNA. • The nuclear genome refers to the DNA in the chromosomes contained in the nucleus; in the case of humans the DNA in the 46 chromosomes. It is the nuclear genome that defines a multicellular organism; it will be the same for all (almost) cells of the organism.
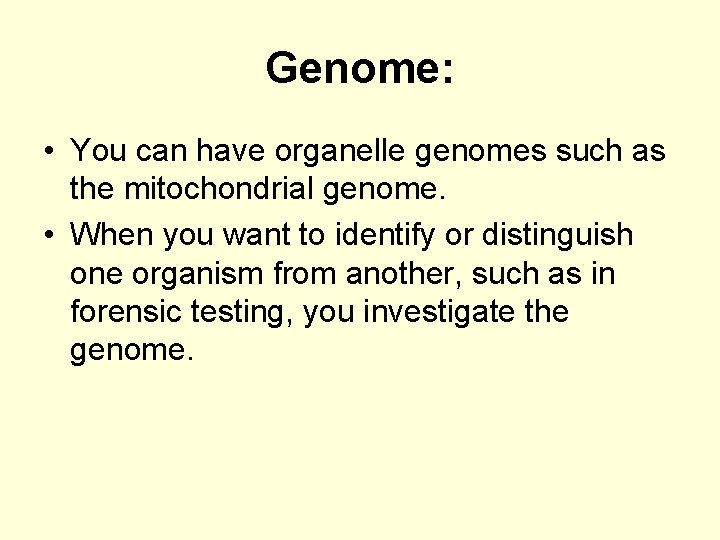
Genome: • You can have organelle genomes such as the mitochondrial genome. • When you want to identify or distinguish one organism from another, such as in forensic testing, you investigate the genome.
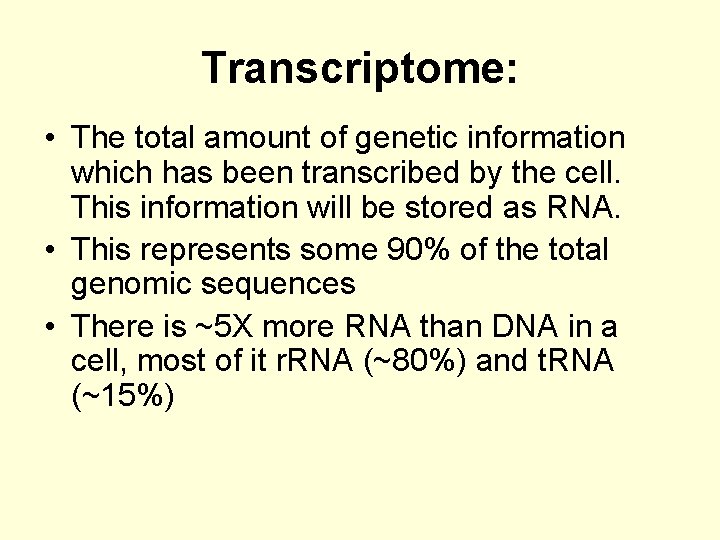
Transcriptome: • The total amount of genetic information which has been transcribed by the cell. This information will be stored as RNA. • This represents some 90% of the total genomic sequences • There is ~5 X more RNA than DNA in a cell, most of it r. RNA (~80%) and t. RNA (~15%)
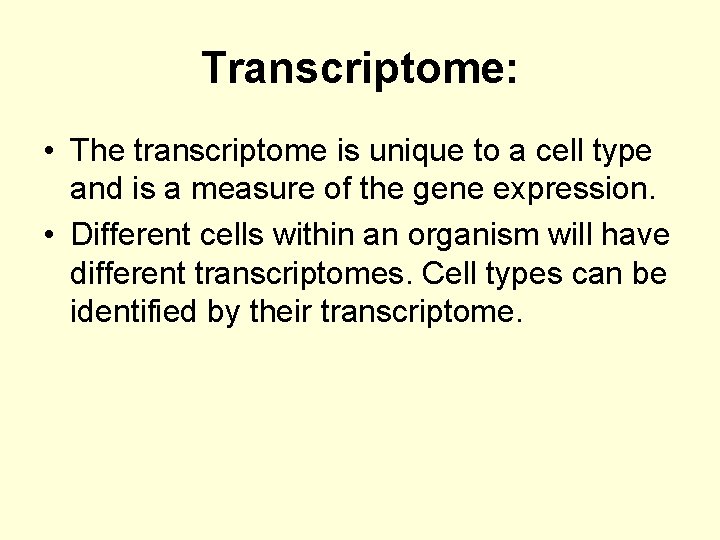
Transcriptome: • The transcriptome is unique to a cell type and is a measure of the gene expression. • Different cells within an organism will have different transcriptomes. Cell types can be identified by their transcriptome.
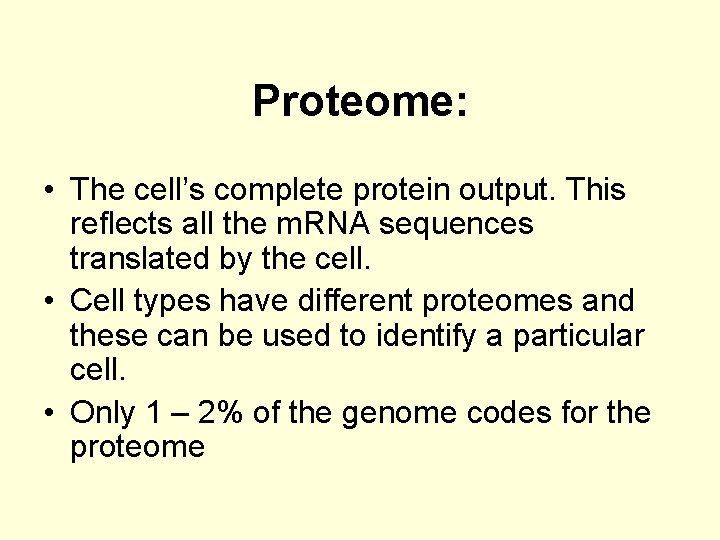
Proteome: • The cell’s complete protein output. This reflects all the m. RNA sequences translated by the cell. • Cell types have different proteomes and these can be used to identify a particular cell. • Only 1 – 2% of the genome codes for the proteome
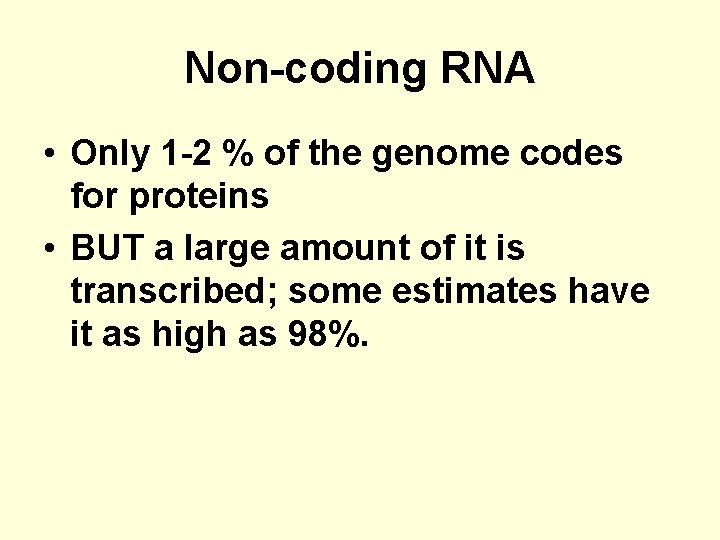
Non-coding RNA • Only 1 -2 % of the genome codes for proteins • BUT a large amount of it is transcribed; some estimates have it as high as 98%.
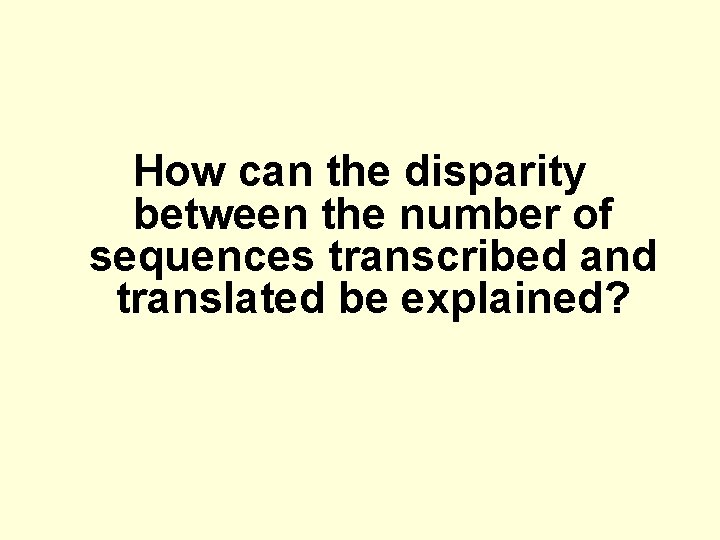
How can the disparity between the number of sequences transcribed and translated be explained?
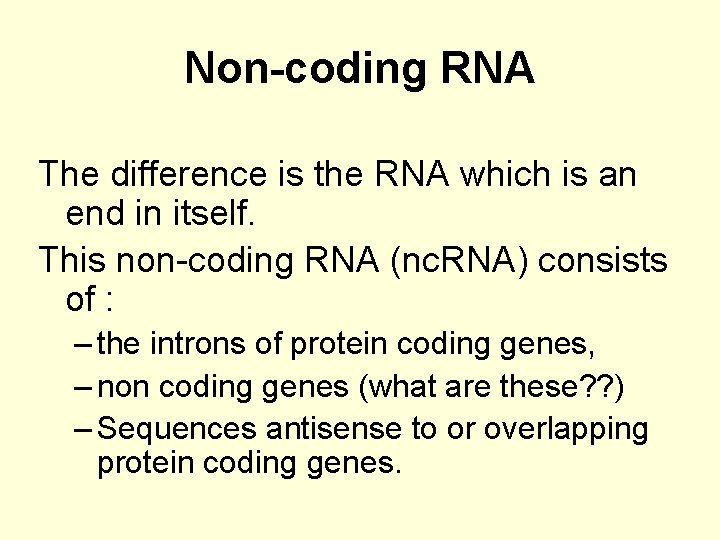
Non-coding RNA The difference is the RNA which is an end in itself. This non-coding RNA (nc. RNA) consists of : – the introns of protein coding genes, – non coding genes (what are these? ? ) – Sequences antisense to or overlapping protein coding genes.
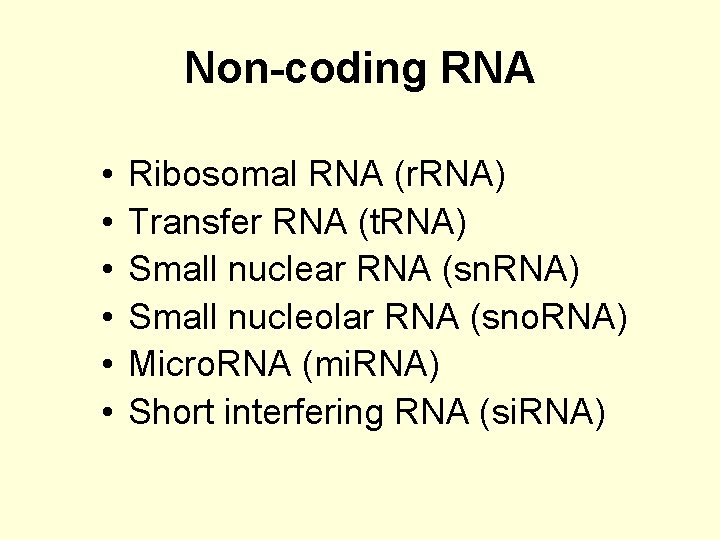
Non-coding RNA • • • Ribosomal RNA (r. RNA) Transfer RNA (t. RNA) Small nuclear RNA (sn. RNA) Small nucleolar RNA (sno. RNA) Micro. RNA (mi. RNA) Short interfering RNA (si. RNA)
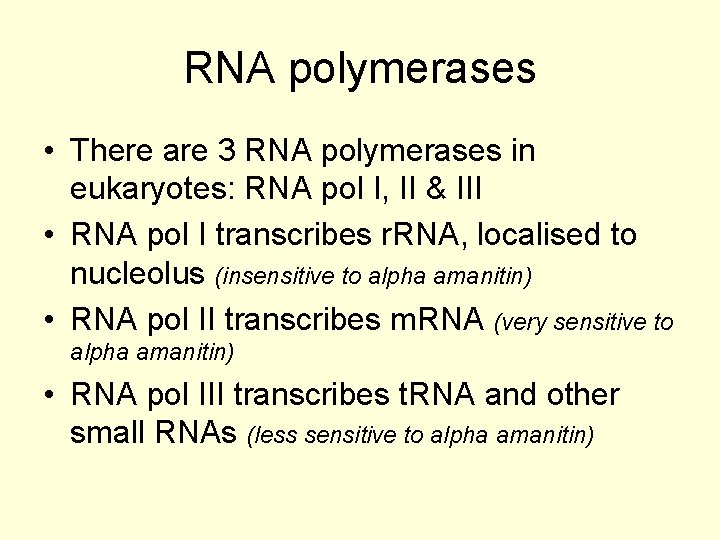
RNA polymerases • There are 3 RNA polymerases in eukaryotes: RNA pol I, II & III • RNA pol I transcribes r. RNA, localised to nucleolus (insensitive to alpha amanitin) • RNA pol II transcribes m. RNA (very sensitive to alpha amanitin) • RNA pol III transcribes t. RNA and other small RNAs (less sensitive to alpha amanitin)
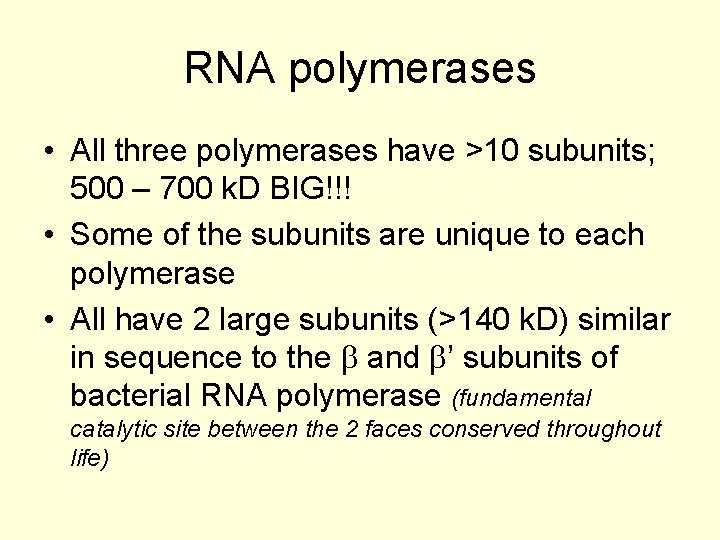
RNA polymerases • All three polymerases have >10 subunits; 500 – 700 k. D BIG!!! • Some of the subunits are unique to each polymerase • All have 2 large subunits (>140 k. D) similar in sequence to the b and b’ subunits of bacterial RNA polymerase (fundamental catalytic site between the 2 faces conserved throughout life)
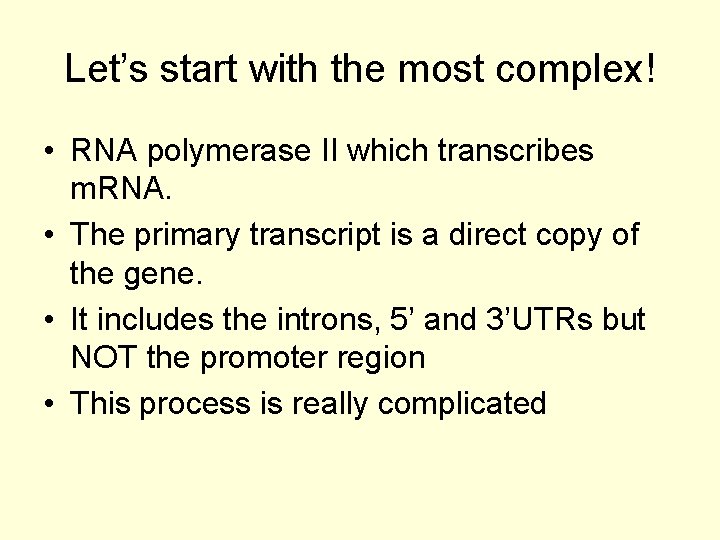
Let’s start with the most complex! • RNA polymerase II which transcribes m. RNA. • The primary transcript is a direct copy of the gene. • It includes the introns, 5’ and 3’UTRs but NOT the promoter region • This process is really complicated
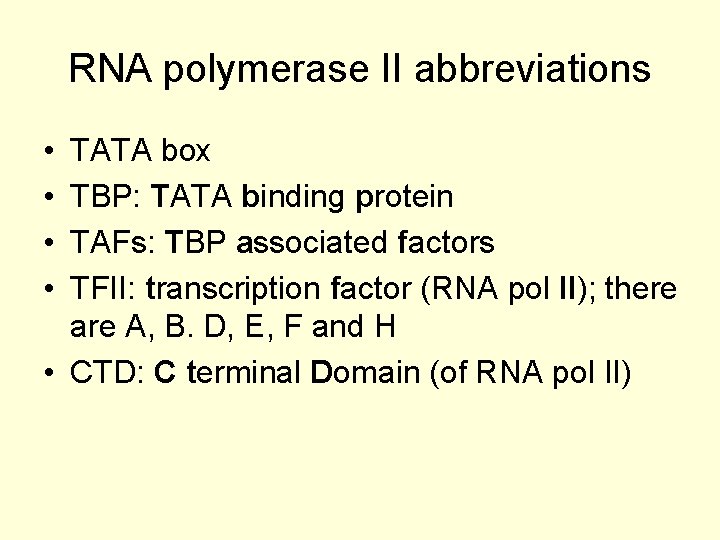
RNA polymerase II abbreviations • • TATA box TBP: TATA binding protein TAFs: TBP associated factors TFII: transcription factor (RNA pol II); there are A, B. D, E, F and H • CTD: C terminal Domain (of RNA pol II)
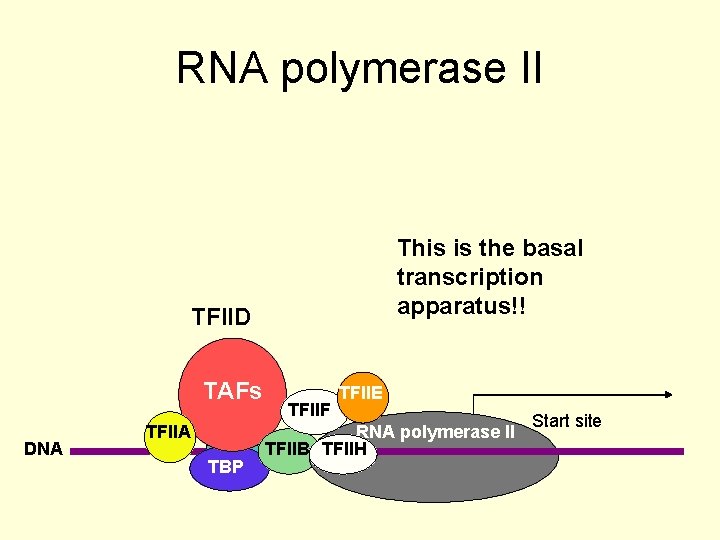
RNA polymerase II This is the basal transcription apparatus!! TFIID TAFs DNA TFIIA TATA TBP TFIIF TFIIE RNA polymerase II TFIIB TFIIH Start site
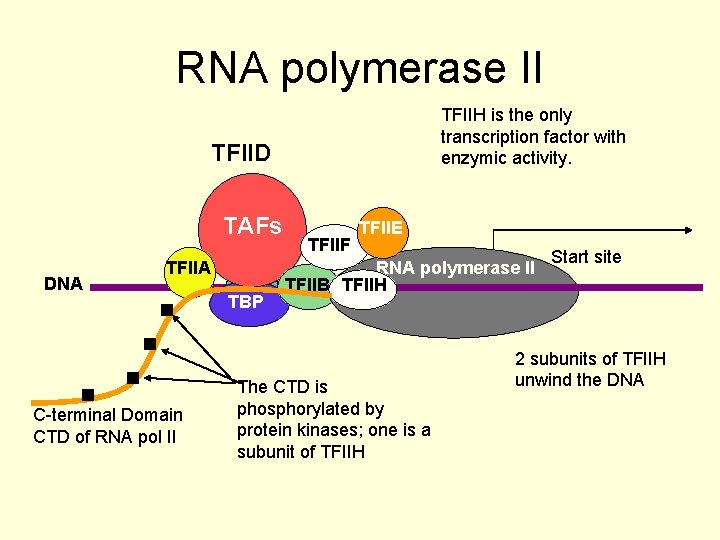
RNA polymerase II TFIIH is the only transcription factor with enzymic activity. TFIID TAFs DNA TFIIA TATA C-terminal Domain CTD of RNA pol II TBP TFIIF TFIIE RNA polymerase II TFIIB TFIIH The CTD is phosphorylated by protein kinases; one is a subunit of TFIIH Start site 2 subunits of TFIIH unwind the DNA
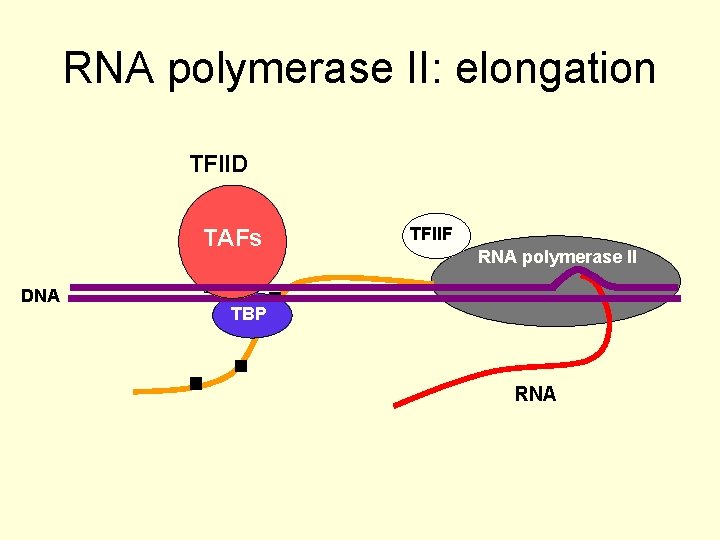
RNA polymerase II: elongation TFIID TAFs TATA DNA TFIIF RNA polymerase II TBP RNA
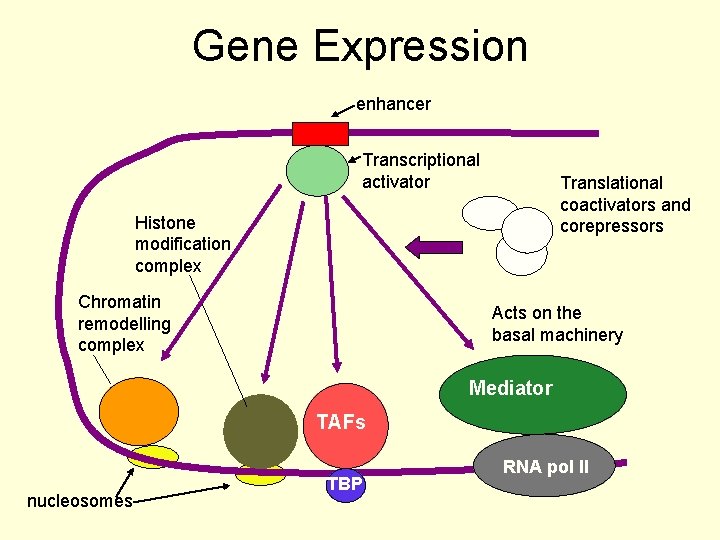
Gene Expression enhancer Transcriptional activator Translational coactivators and corepressors Histone modification complex Chromatin remodelling complex Acts on the basal machinery Mediator TAFs nucleosomes TBP RNA pol II
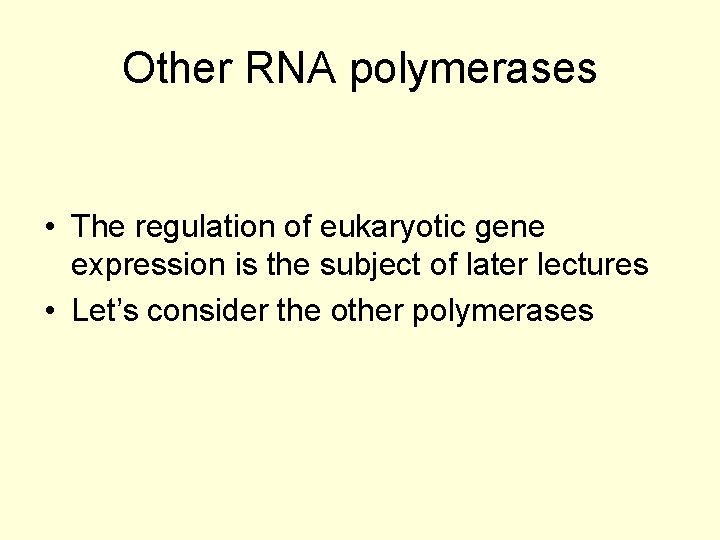
Other RNA polymerases • The regulation of eukaryotic gene expression is the subject of later lectures • Let’s consider the other polymerases
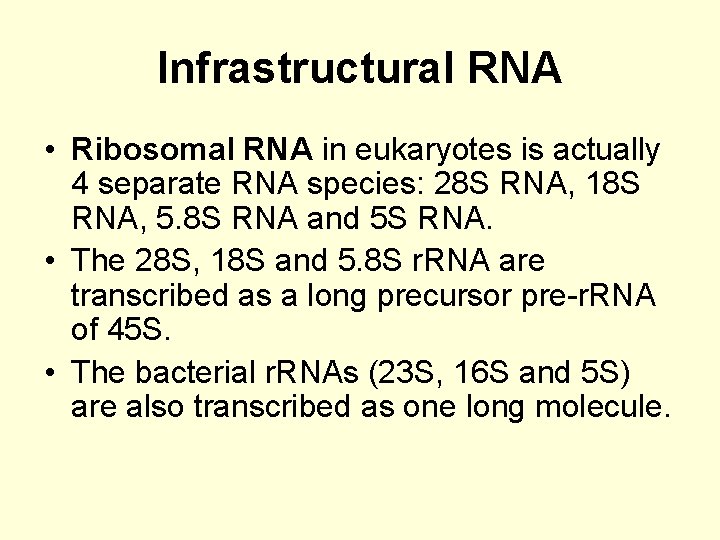
Infrastructural RNA • Ribosomal RNA in eukaryotes is actually 4 separate RNA species: 28 S RNA, 18 S RNA, 5. 8 S RNA and 5 S RNA. • The 28 S, 18 S and 5. 8 S r. RNA are transcribed as a long precursor pre-r. RNA of 45 S. • The bacterial r. RNAs (23 S, 16 S and 5 S) are also transcribed as one long molecule.
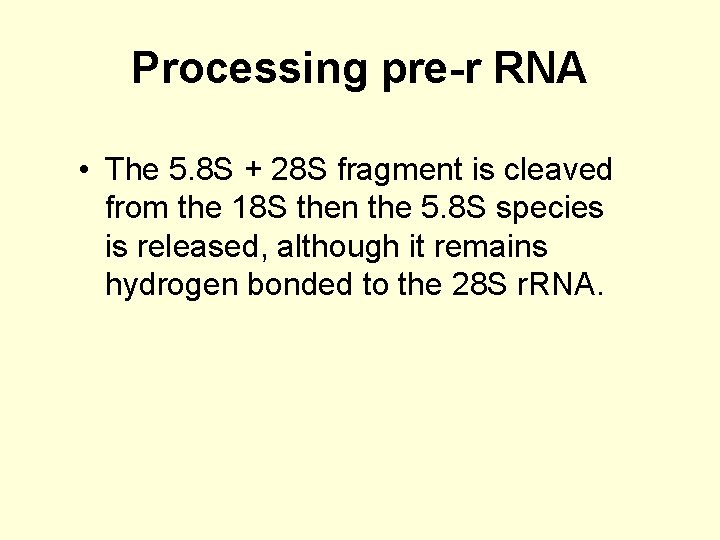
Processing pre-r RNA • The 5. 8 S + 28 S fragment is cleaved from the 18 S then the 5. 8 S species is released, although it remains hydrogen bonded to the 28 S r. RNA.
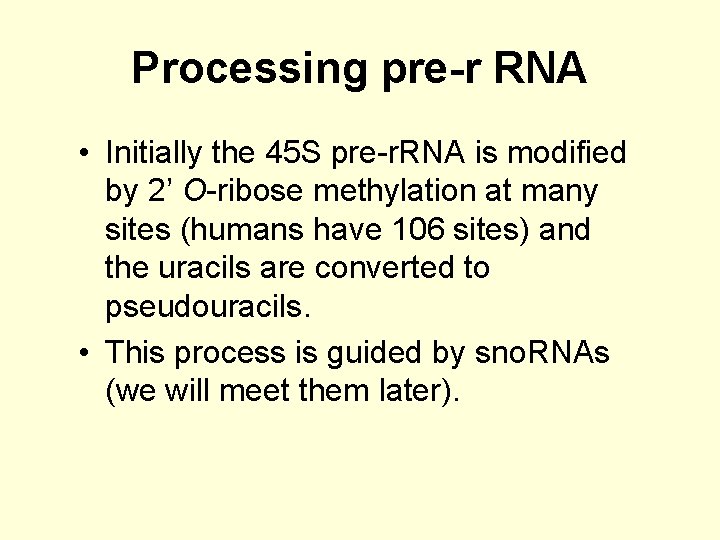
Processing pre-r RNA • Initially the 45 S pre-r. RNA is modified by 2’ O-ribose methylation at many sites (humans have 106 sites) and the uracils are converted to pseudouracils. • This process is guided by sno. RNAs (we will meet them later).
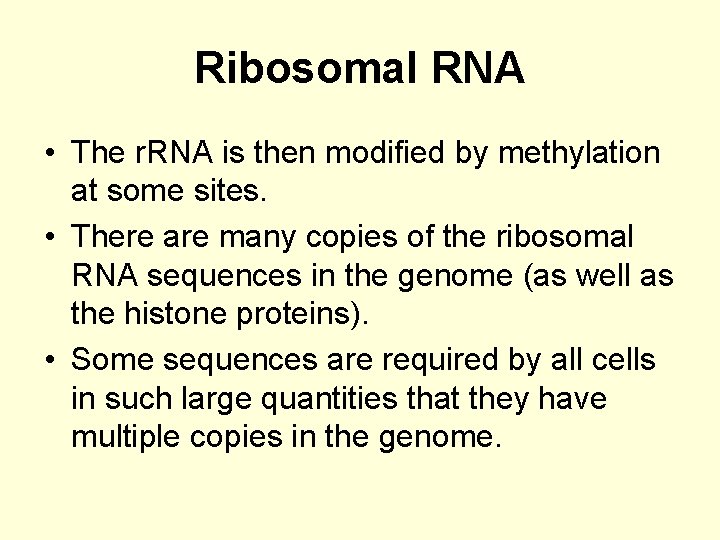
Ribosomal RNA • The r. RNA is then modified by methylation at some sites. • There are many copies of the ribosomal RNA sequences in the genome (as well as the histone proteins). • Some sequences are required by all cells in such large quantities that they have multiple copies in the genome.
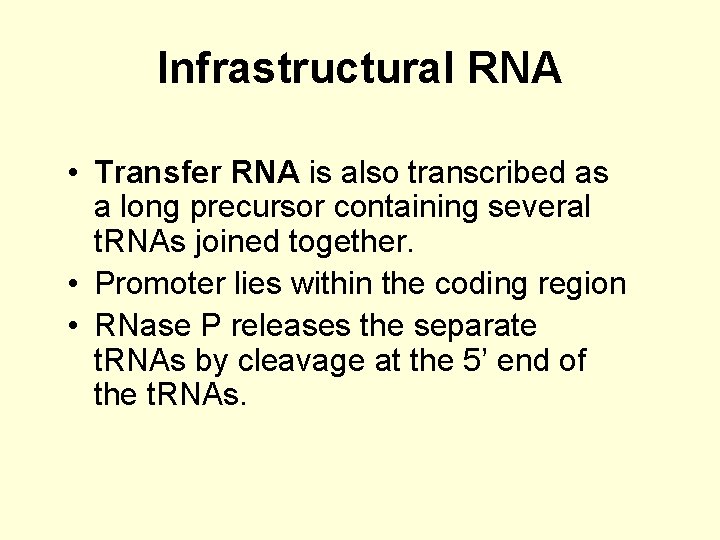
Infrastructural RNA • Transfer RNA is also transcribed as a long precursor containing several t. RNAs joined together. • Promoter lies within the coding region • RNase P releases the separate t. RNAs by cleavage at the 5’ end of the t. RNAs.
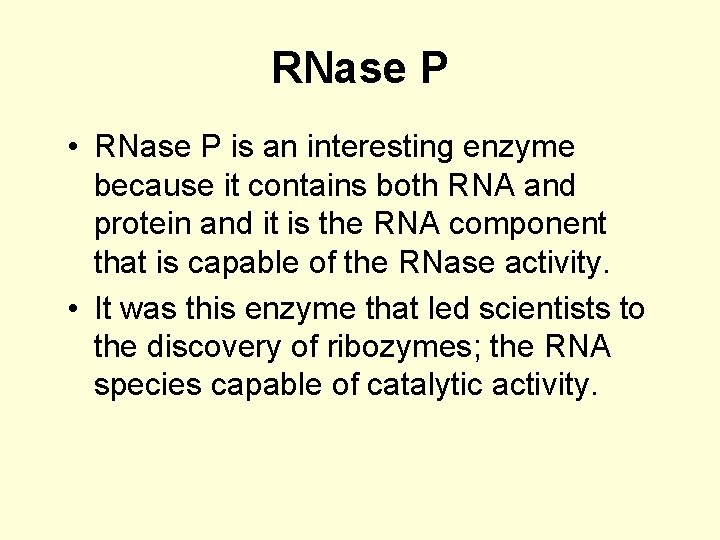
RNase P • RNase P is an interesting enzyme because it contains both RNA and protein and it is the RNA component that is capable of the RNase activity. • It was this enzyme that led scientists to the discovery of ribozymes; the RNA species capable of catalytic activity.
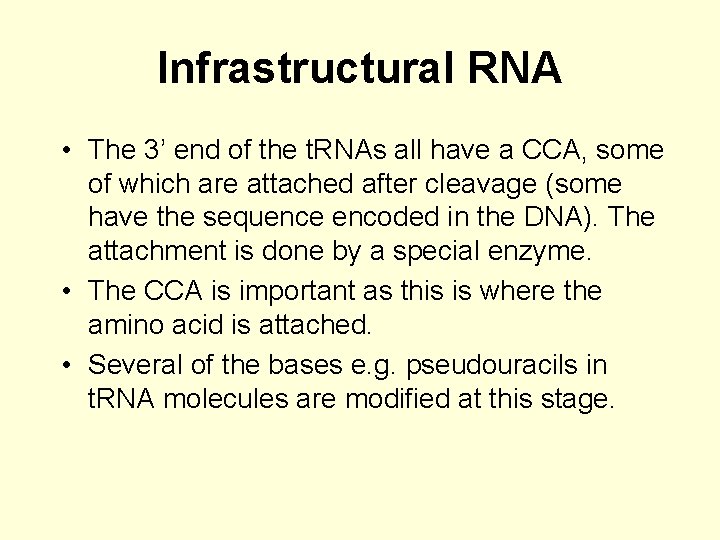
Infrastructural RNA • The 3’ end of the t. RNAs all have a CCA, some of which are attached after cleavage (some have the sequence encoded in the DNA). The attachment is done by a special enzyme. • The CCA is important as this is where the amino acid is attached. • Several of the bases e. g. pseudouracils in t. RNA molecules are modified at this stage.
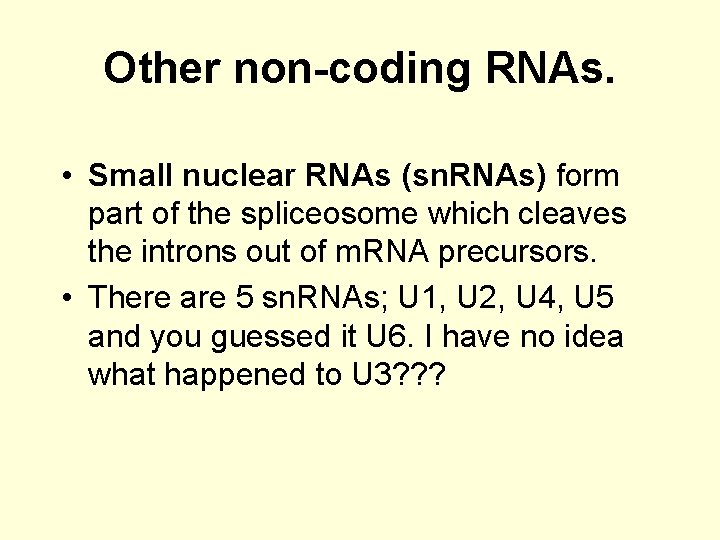
Other non-coding RNAs. • Small nuclear RNAs (sn. RNAs) form part of the spliceosome which cleaves the introns out of m. RNA precursors. • There are 5 sn. RNAs; U 1, U 2, U 4, U 5 and you guessed it U 6. I have no idea what happened to U 3? ? ?
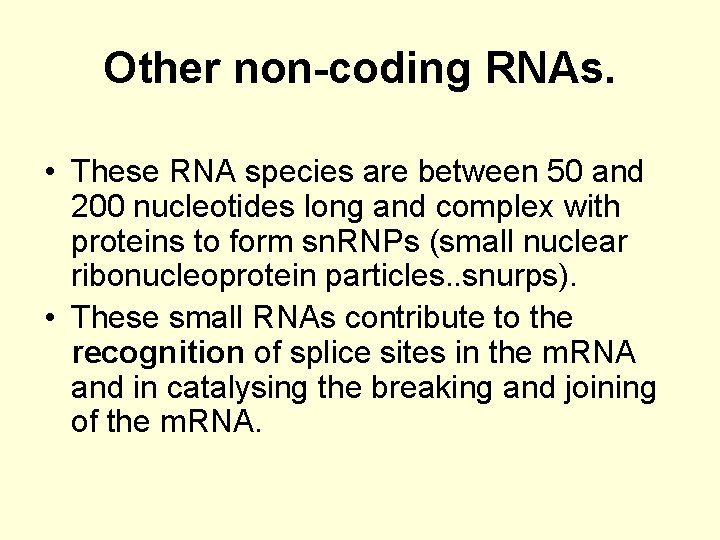
Other non-coding RNAs. • These RNA species are between 50 and 200 nucleotides long and complex with proteins to form sn. RNPs (small nuclear ribonucleoprotein particles. . snurps). • These small RNAs contribute to the recognition of splice sites in the m. RNA and in catalysing the breaking and joining of the m. RNA.
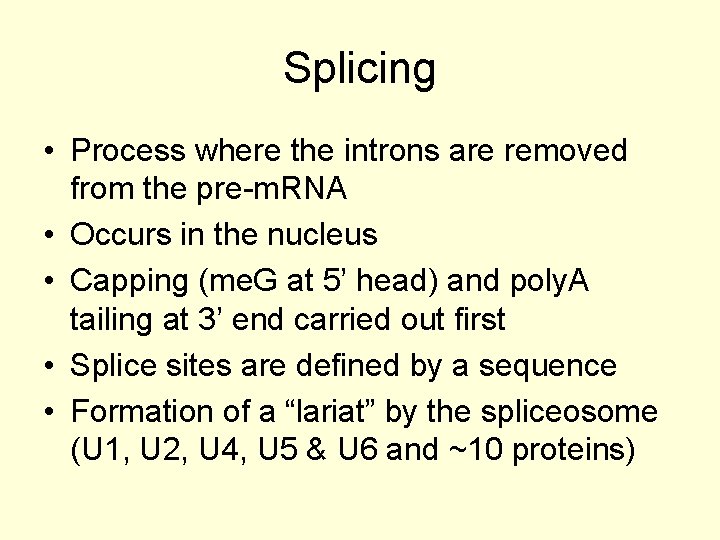
Splicing • Process where the introns are removed from the pre-m. RNA • Occurs in the nucleus • Capping (me. G at 5’ head) and poly. A tailing at 3’ end carried out first • Splice sites are defined by a sequence • Formation of a “lariat” by the spliceosome (U 1, U 2, U 4, U 5 & U 6 and ~10 proteins)
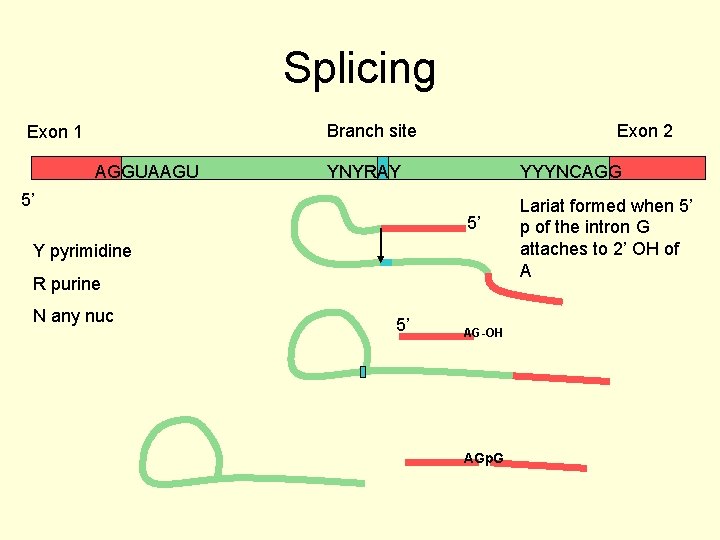
Splicing Branch site Exon 1 AGGUAAGU Exon 2 YNYRAY YYYNCAGG 5’ 5’ Y pyrimidine R purine N any nuc 5’ AG-OH AGp. G Lariat formed when 5’ p of the intron G attaches to 2’ OH of A
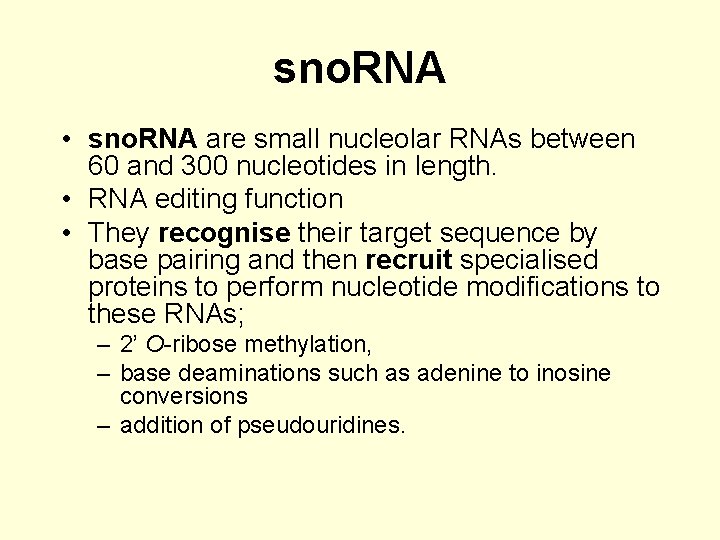
sno. RNA • sno. RNA are small nucleolar RNAs between 60 and 300 nucleotides in length. • RNA editing function • They recognise their target sequence by base pairing and then recruit specialised proteins to perform nucleotide modifications to these RNAs; – 2’ O-ribose methylation, – base deaminations such as adenine to inosine conversions – addition of pseudouridines.
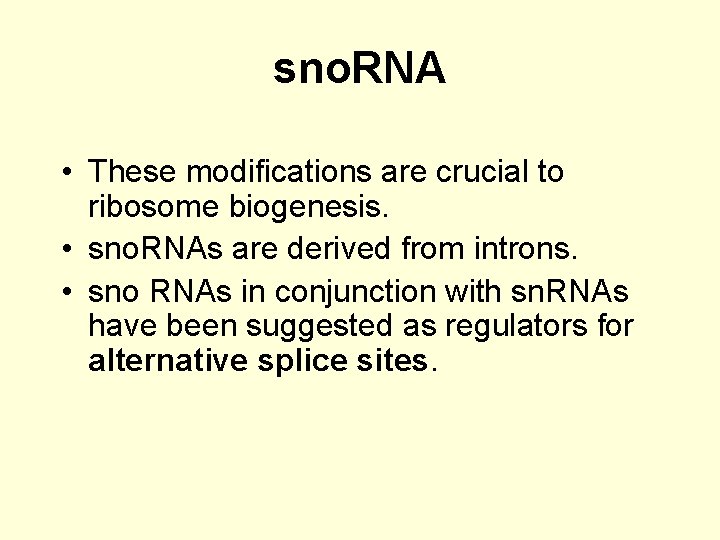
sno. RNA • These modifications are crucial to ribosome biogenesis. • sno. RNAs are derived from introns. • sno RNAs in conjunction with sn. RNAs have been suggested as regulators for alternative splice sites.
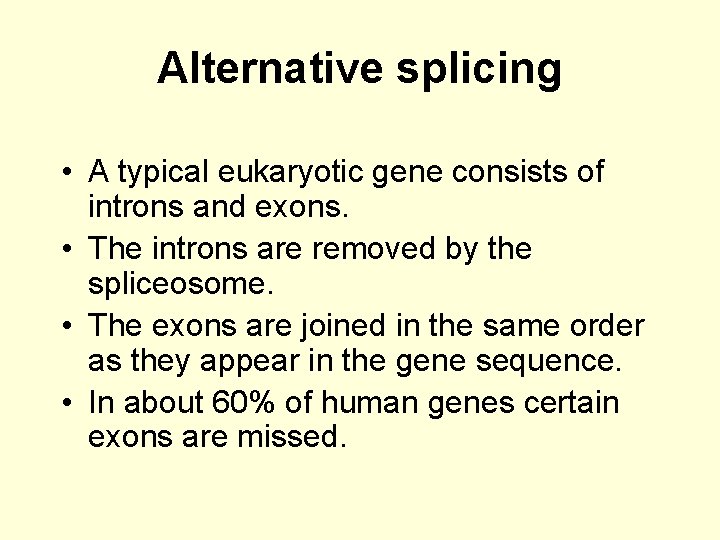
Alternative splicing • A typical eukaryotic gene consists of introns and exons. • The introns are removed by the spliceosome. • The exons are joined in the same order as they appear in the gene sequence. • In about 60% of human genes certain exons are missed.
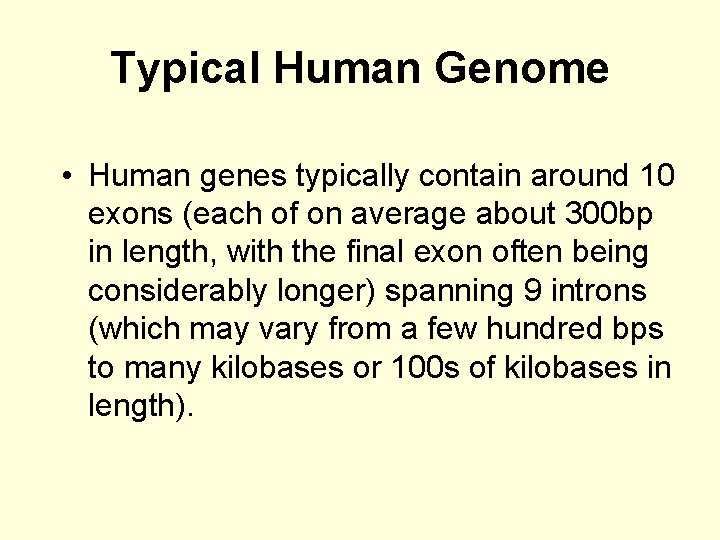
Typical Human Genome • Human genes typically contain around 10 exons (each of on average about 300 bp in length, with the final exon often being considerably longer) spanning 9 introns (which may vary from a few hundred bps to many kilobases or 100 s of kilobases in length).
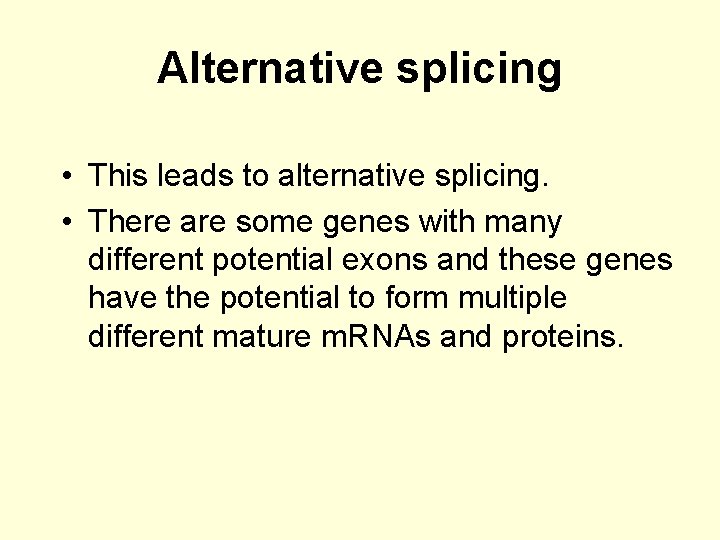
Alternative splicing • This leads to alternative splicing. • There are some genes with many different potential exons and these genes have the potential to form multiple different mature m. RNAs and proteins.
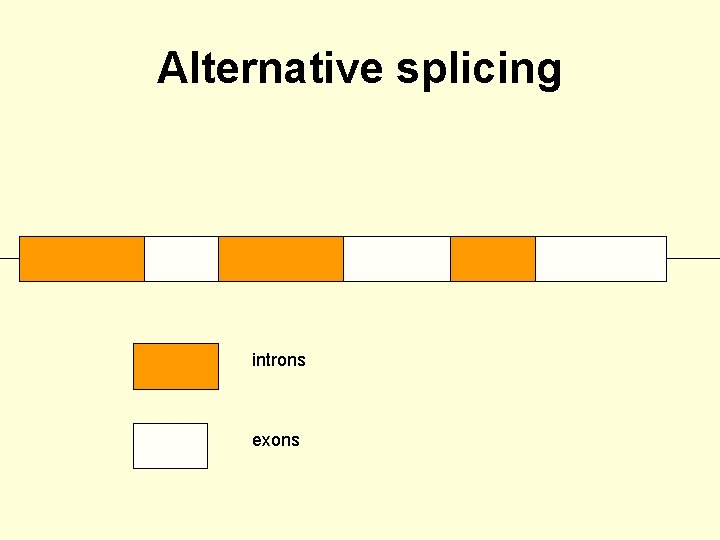
Alternative splicing introns exons
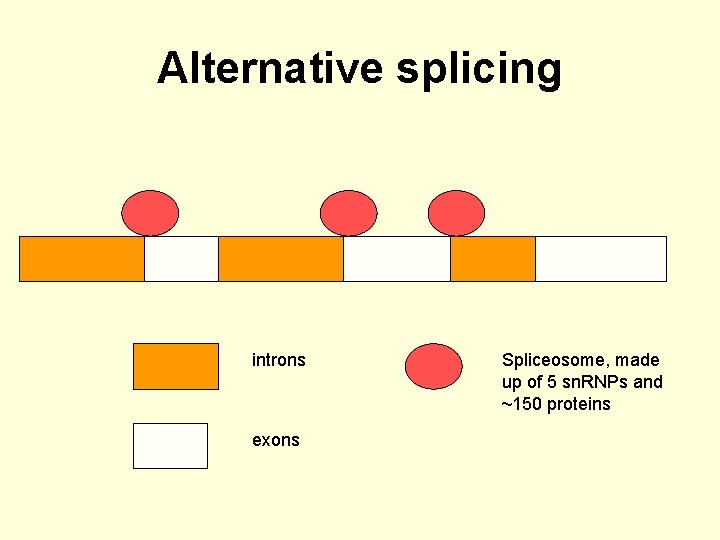
Alternative splicing introns exons Spliceosome, made up of 5 sn. RNPs and ~150 proteins
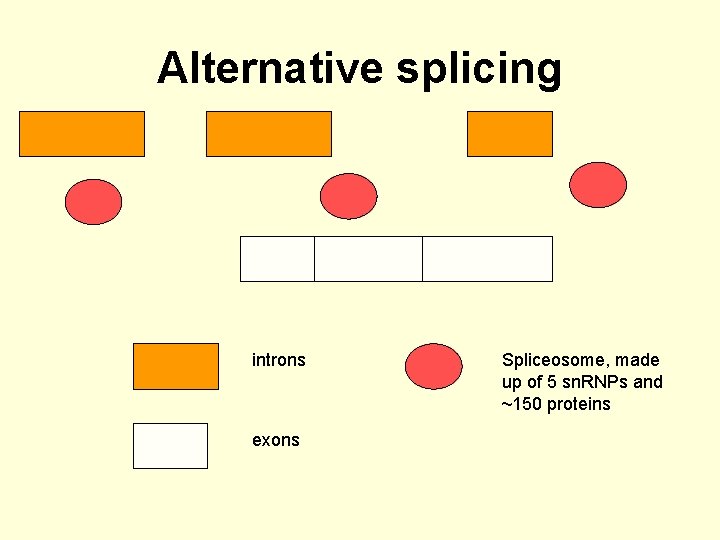
Alternative splicing introns exons Spliceosome, made up of 5 sn. RNPs and ~150 proteins
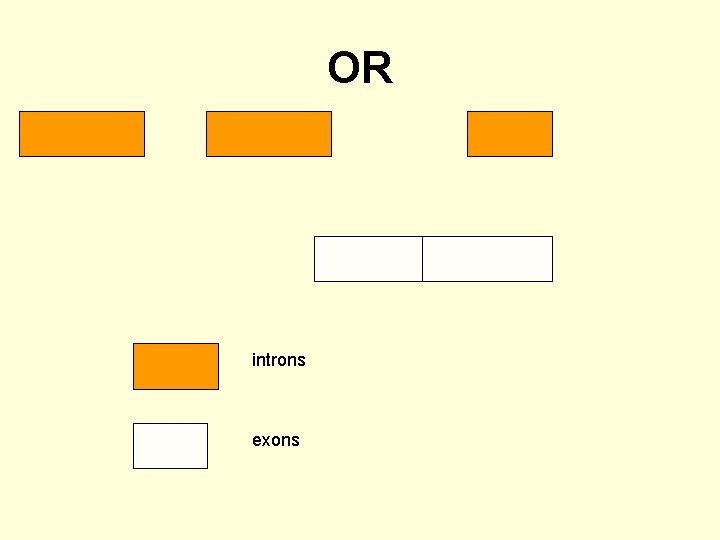
OR introns exons
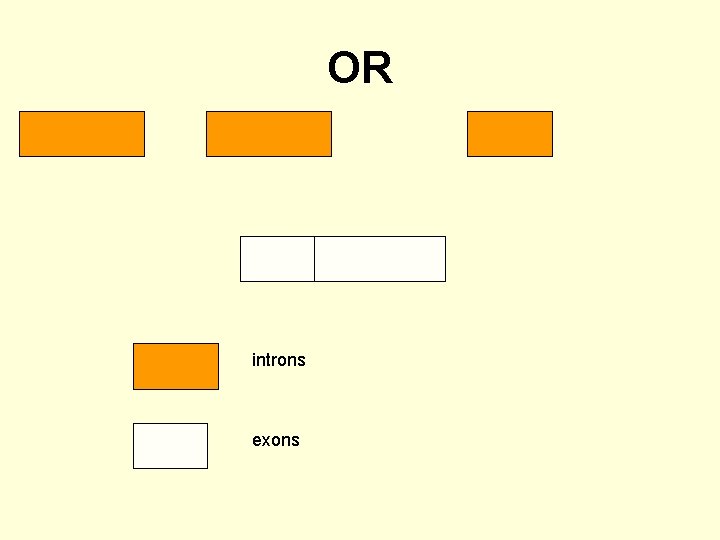
OR introns exons
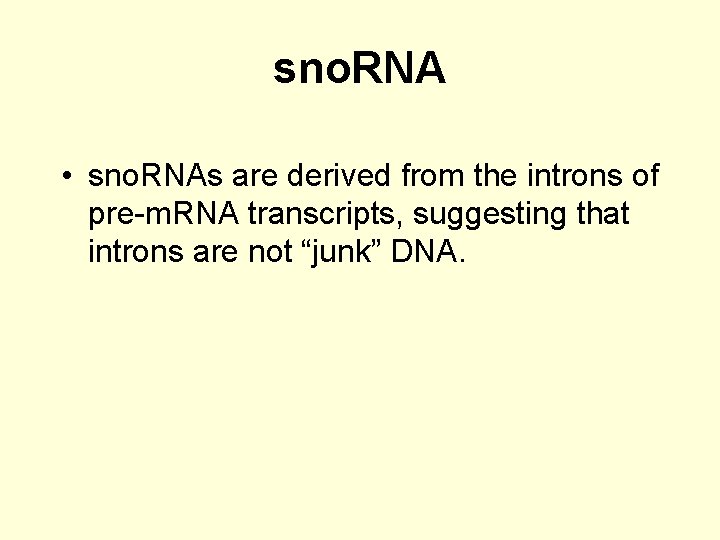
sno. RNA • sno. RNAs are derived from the introns of pre-m. RNA transcripts, suggesting that introns are not “junk” DNA.
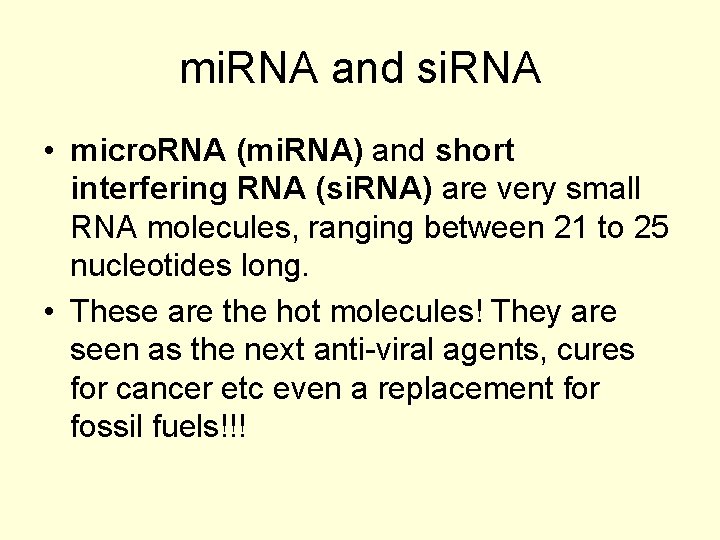
mi. RNA and si. RNA • micro. RNA (mi. RNA) and short interfering RNA (si. RNA) are very small RNA molecules, ranging between 21 to 25 nucleotides long. • These are the hot molecules! They are seen as the next anti-viral agents, cures for cancer etc even a replacement for fossil fuels!!!
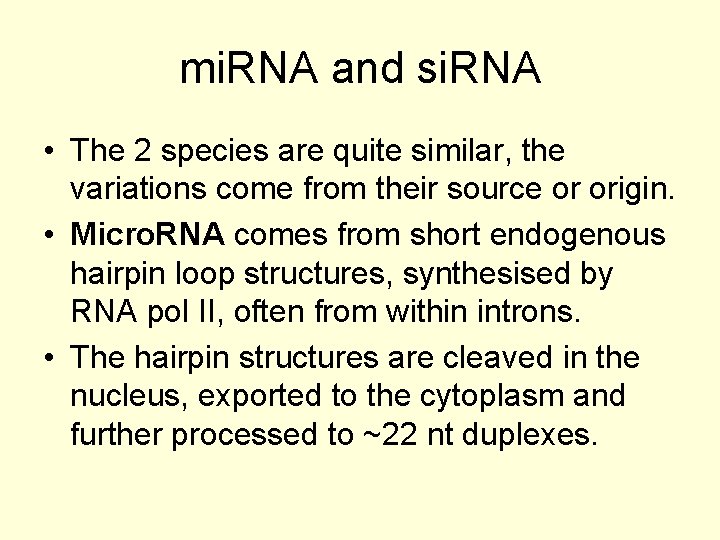
mi. RNA and si. RNA • The 2 species are quite similar, the variations come from their source or origin. • Micro. RNA comes from short endogenous hairpin loop structures, synthesised by RNA pol II, often from within introns. • The hairpin structures are cleaved in the nucleus, exported to the cytoplasm and further processed to ~22 nt duplexes.
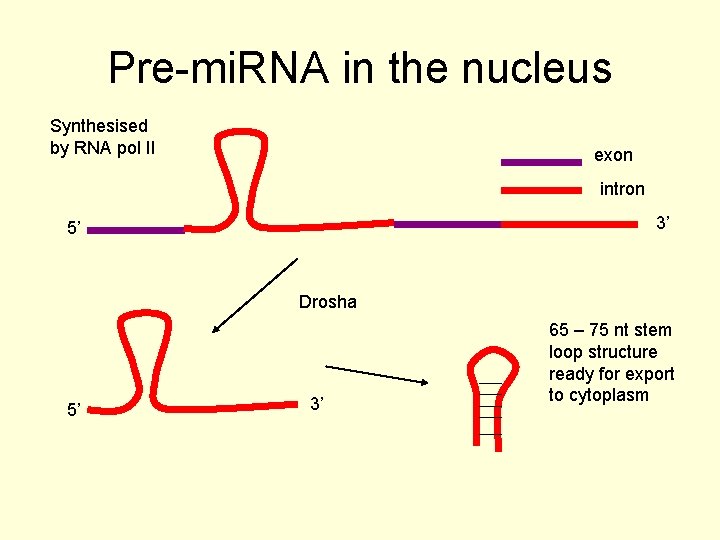
Pre-mi. RNA in the nucleus Synthesised by RNA pol II exon intron 3’ 5’ Drosha 5’ 3’ 65 – 75 nt stem loop structure ready for export to cytoplasm
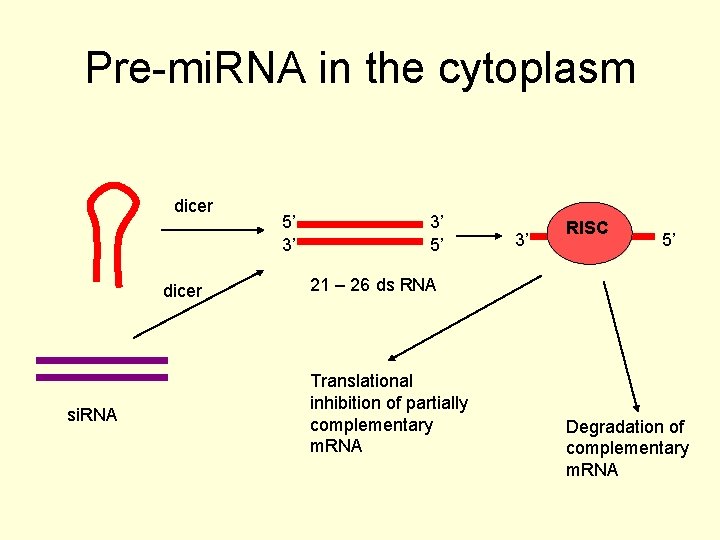
Pre-mi. RNA in the cytoplasm dicer si. RNA 5’ 3’ 3’ 5’ 3’ RISC 5’ 21 – 26 ds RNA Translational inhibition of partially complementary m. RNA Degradation of complementary m. RNA
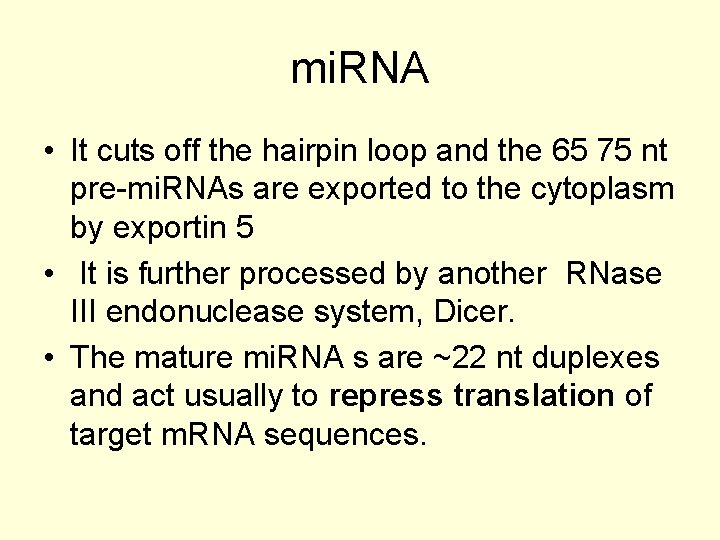
mi. RNA • It cuts off the hairpin loop and the 65 75 nt pre-mi. RNAs are exported to the cytoplasm by exportin 5 • It is further processed by another RNase III endonuclease system, Dicer. • The mature mi. RNA s are ~22 nt duplexes and act usually to repress translation of target m. RNA sequences.
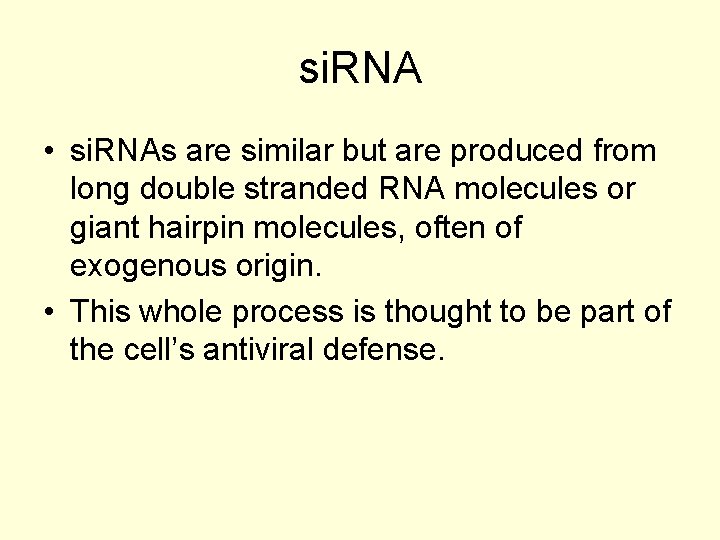
si. RNA • si. RNAs are similar but are produced from long double stranded RNA molecules or giant hairpin molecules, often of exogenous origin. • This whole process is thought to be part of the cell’s antiviral defense.
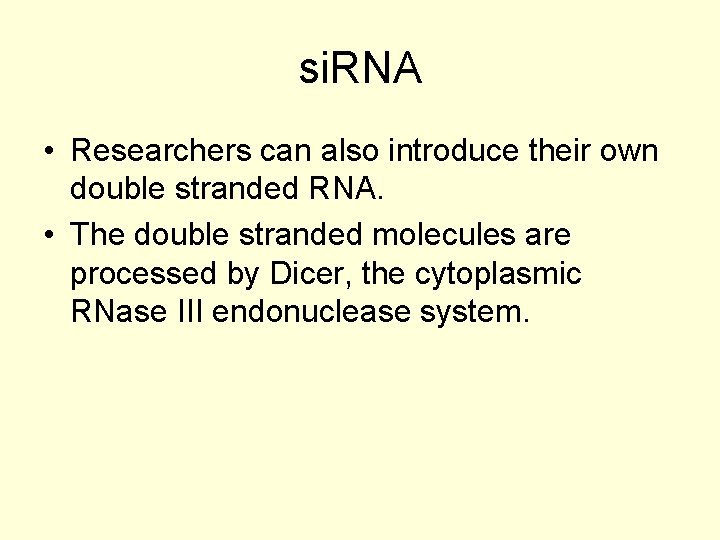
si. RNA • Researchers can also introduce their own double stranded RNA. • The double stranded molecules are processed by Dicer, the cytoplasmic RNase III endonuclease system.
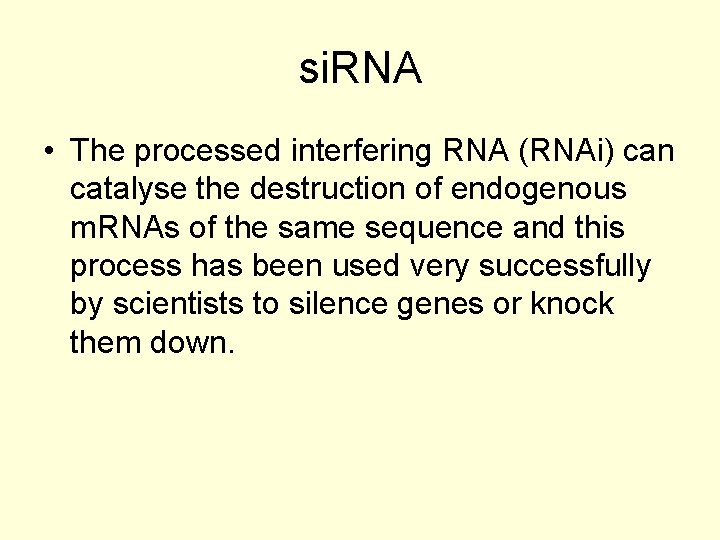
si. RNA • The processed interfering RNA (RNAi) can catalyse the destruction of endogenous m. RNAs of the same sequence and this process has been used very successfully by scientists to silence genes or knock them down.
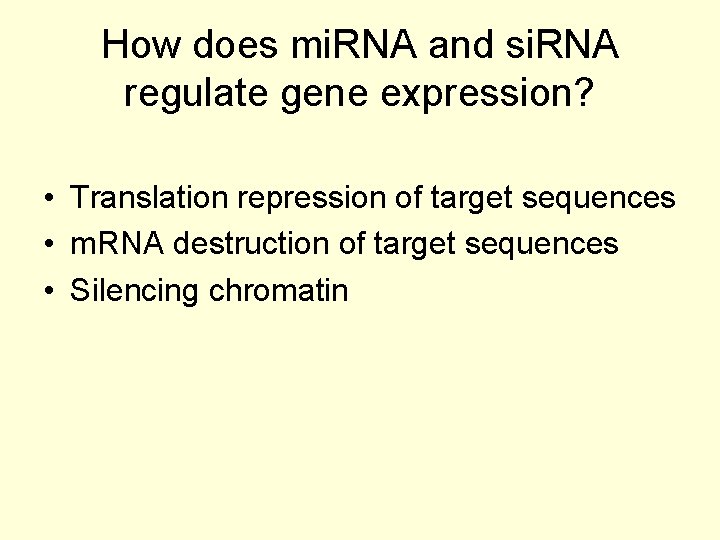
How does mi. RNA and si. RNA regulate gene expression? • Translation repression of target sequences • m. RNA destruction of target sequences • Silencing chromatin
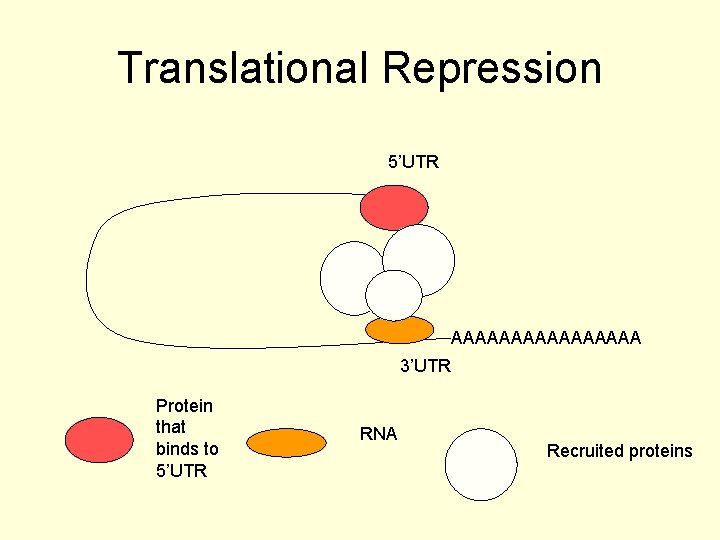
Translational Repression 5’UTR AAAAAAAA 3’UTR Protein that binds to 5’UTR RNA Recruited proteins
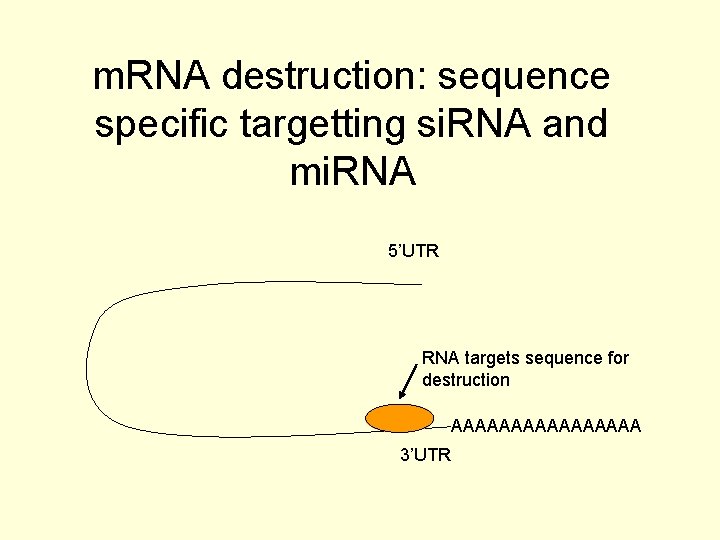
m. RNA destruction: sequence specific targetting si. RNA and mi. RNA 5’UTR RNA targets sequence for destruction AAAAAAAA 3’UTR
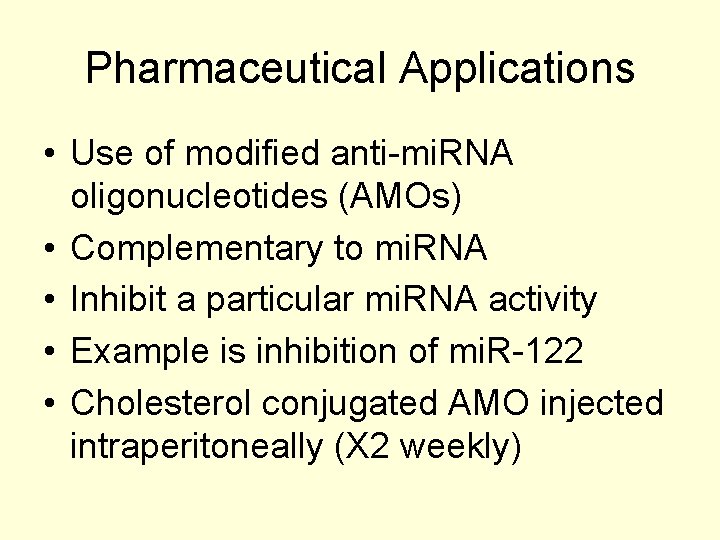
Pharmaceutical Applications • Use of modified anti-mi. RNA oligonucleotides (AMOs) • Complementary to mi. RNA • Inhibit a particular mi. RNA activity • Example is inhibition of mi. R-122 • Cholesterol conjugated AMO injected intraperitoneally (X 2 weekly)
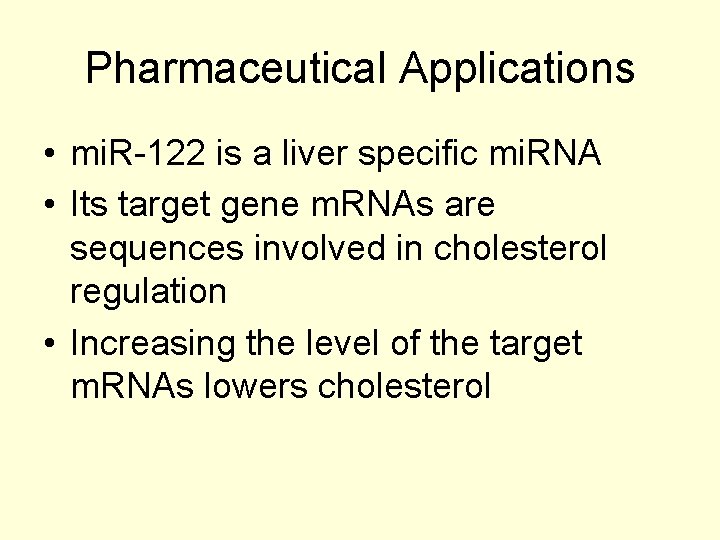
Pharmaceutical Applications • mi. R-122 is a liver specific mi. RNA • Its target gene m. RNAs are sequences involved in cholesterol regulation • Increasing the level of the target m. RNAs lowers cholesterol
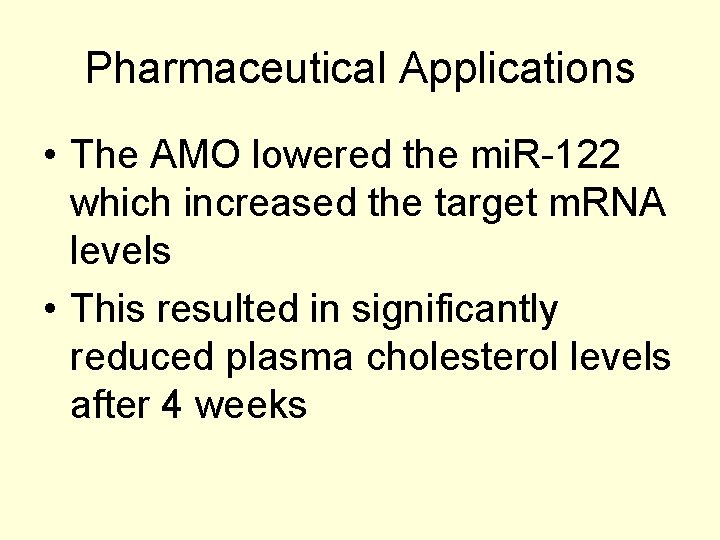
Pharmaceutical Applications • The AMO lowered the mi. R-122 which increased the target m. RNA levels • This resulted in significantly reduced plasma cholesterol levels after 4 weeks
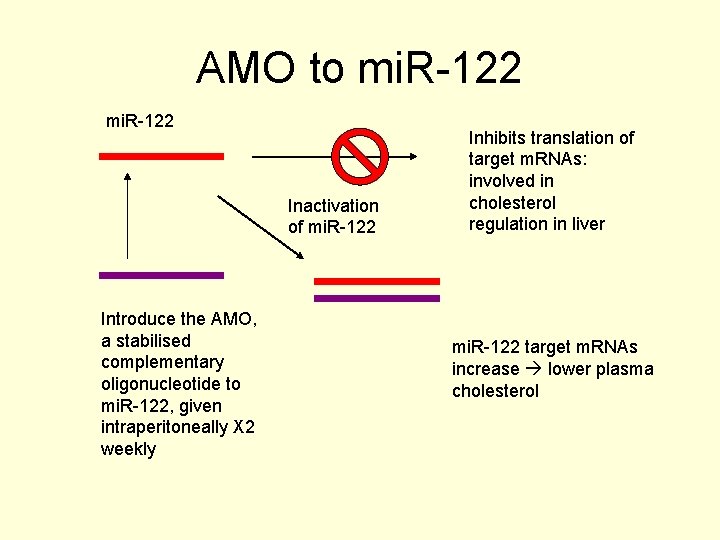
AMO to mi. R-122 Inactivation of mi. R-122 Introduce the AMO, a stabilised complementary oligonucleotide to mi. R-122, given intraperitoneally X 2 weekly Inhibits translation of target m. RNAs: involved in cholesterol regulation in liver mi. R-122 target m. RNAs increase lower plasma cholesterol
Dale's cone of learning
Dales shoes
Dale cone of experience
Thomas hoetzlein
Li ping phar
Ustekinumab
Leo phar
Li ping phar
01:640:244 lecture notes - lecture 15: plat, idah, farad
Chụp phim tư thế worms-breton
đại từ thay thế
Quá trình desamine hóa có thể tạo ra
Công thức tính thế năng
Dạng đột biến một nhiễm là
Bổ thể
Vẽ hình chiếu đứng bằng cạnh của vật thể
Phản ứng thế ankan
Môn thể thao bắt đầu bằng từ chạy
Sơ đồ cơ thể người
Thiếu nhi thế giới liên hoan
điện thế nghỉ
Thế nào là sự mỏi cơ
Một số thể thơ truyền thống
Trời xanh đây là của chúng ta thể thơ
ưu thế lai là gì
Số.nguyên tố
Tỉ lệ cơ thể trẻ em
Fecboak
Các châu lục và đại dương trên thế giới
Thế nào là hệ số cao nhất
Từ ngữ thể hiện lòng nhân hậu
Tư thế ngồi viết
Hát kết hợp bộ gõ cơ thể
đặc điểm cơ thể của người tối cổ
Cái miệng bé xinh thế chỉ nói điều hay thôi
Mật thư anh em như thể tay chân
Khi nào hổ mẹ dạy hổ con săn mồi
Tư thế ngồi viết
Gấu đi như thế nào
Thẻ vin
Thể thơ truyền thống
Các châu lục và đại dương trên thế giới
Thế nào là mạng điện lắp đặt kiểu nổi
Diễn thế sinh thái là
Lp html
Thế nào là giọng cùng tên
Vẽ hình chiếu vuông góc của vật thể sau
101012 bằng
Chúa yêu trần thế
Khi nào hổ con có thể sống độc lập
Lời thề hippocrates
Hamlet plot
Den videnskabelige basismodel
Albrecht durer paradise lost
Synopsis
Gode problemformuleringer eksempler
Skins synopsis