Heat 1 Heat Q is the energy transferred
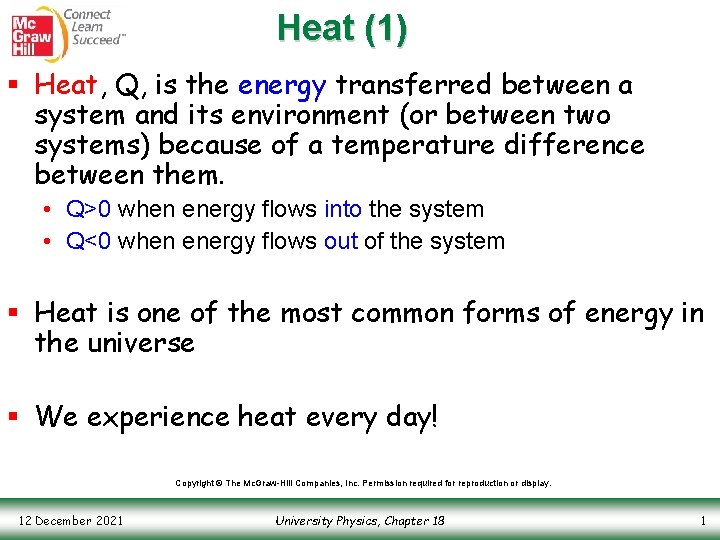
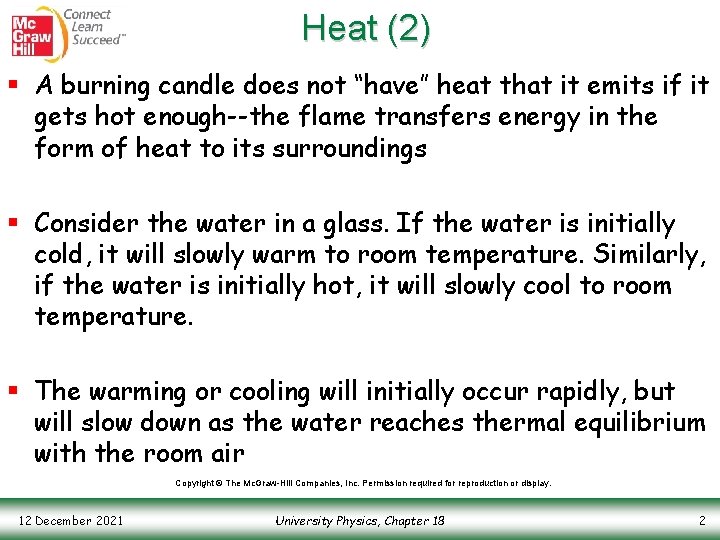
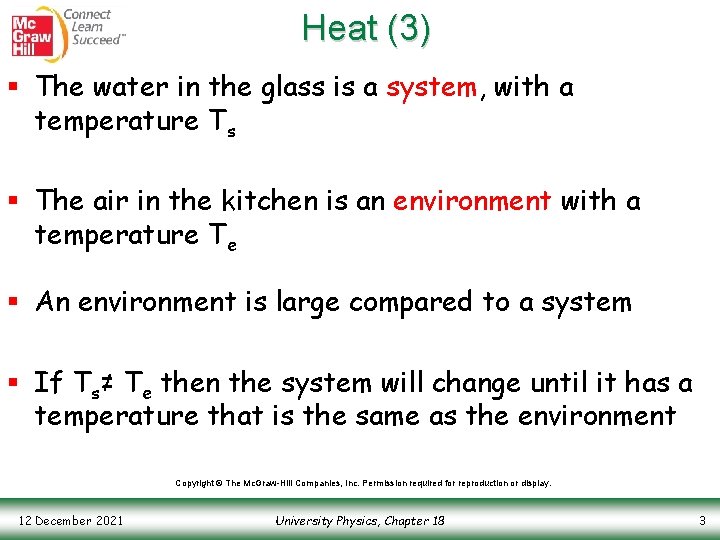
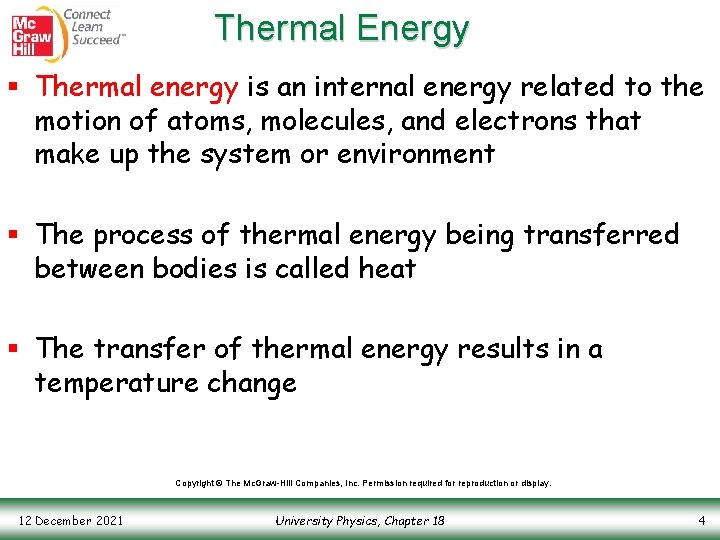
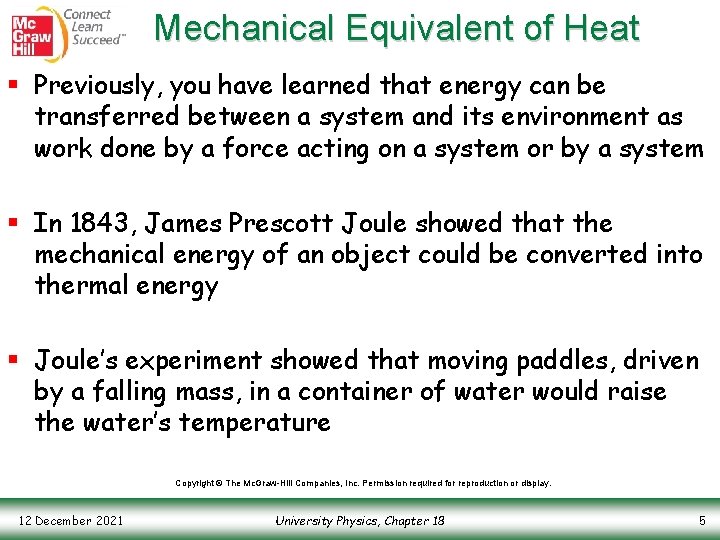
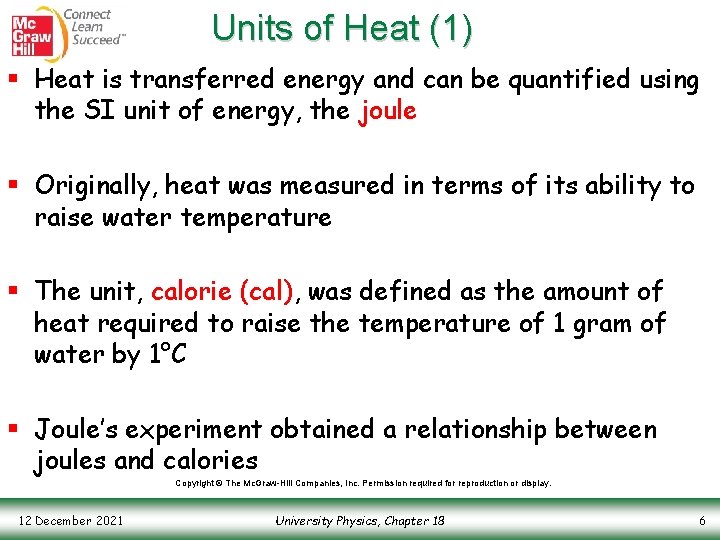
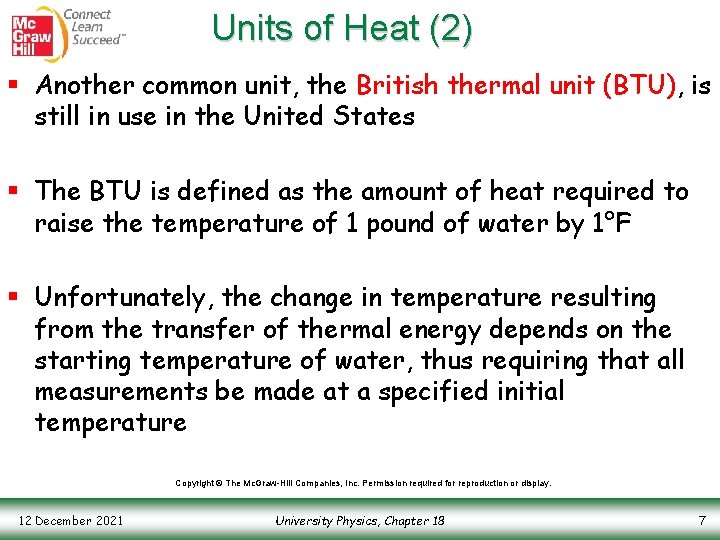
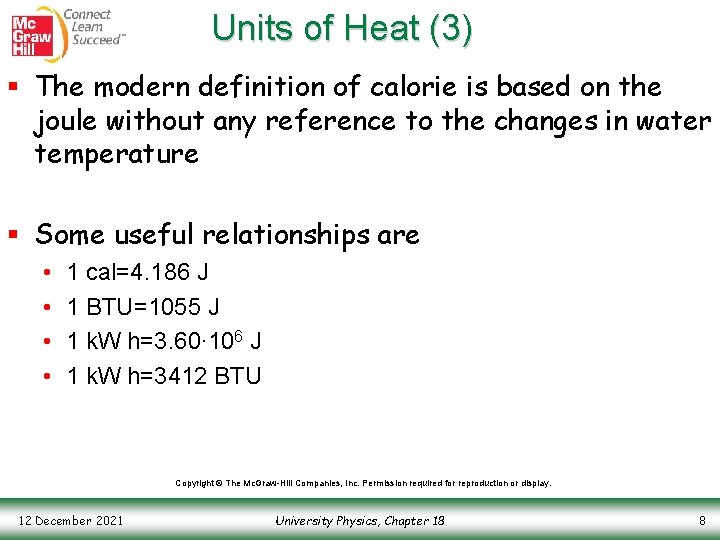
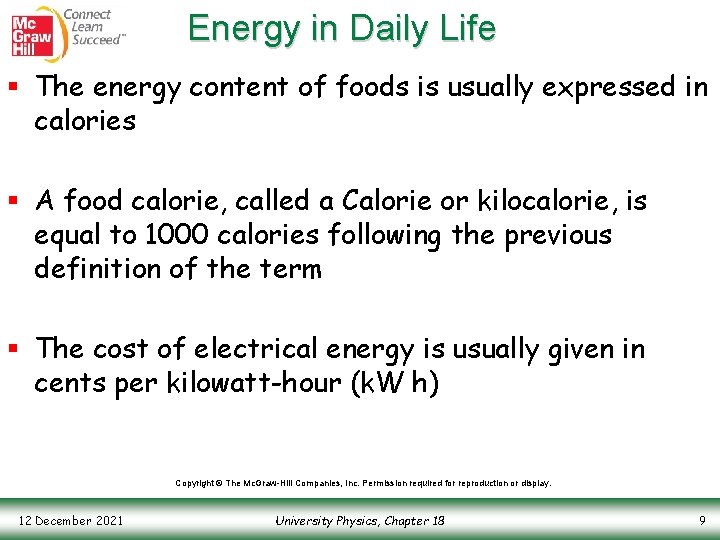
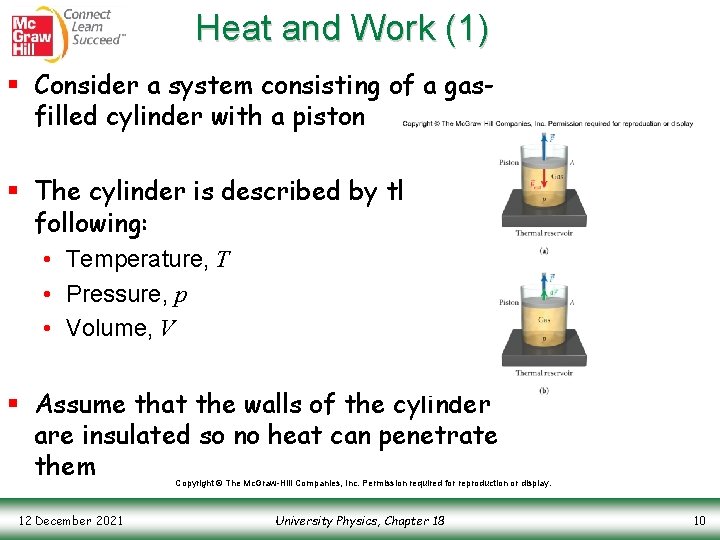
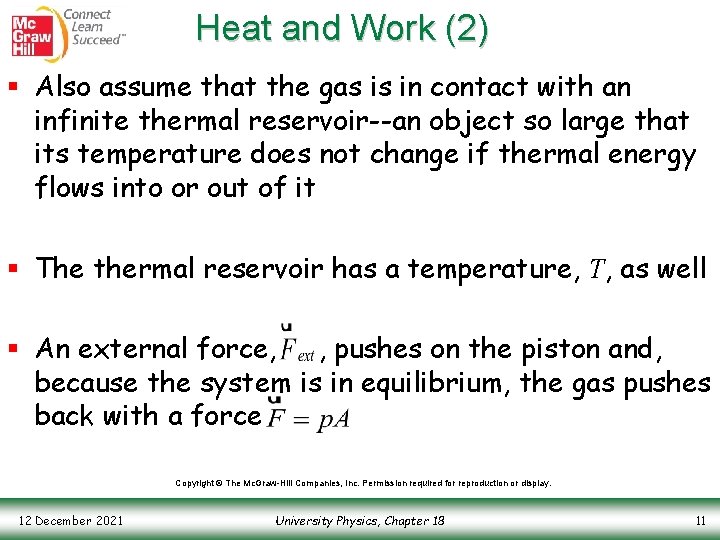
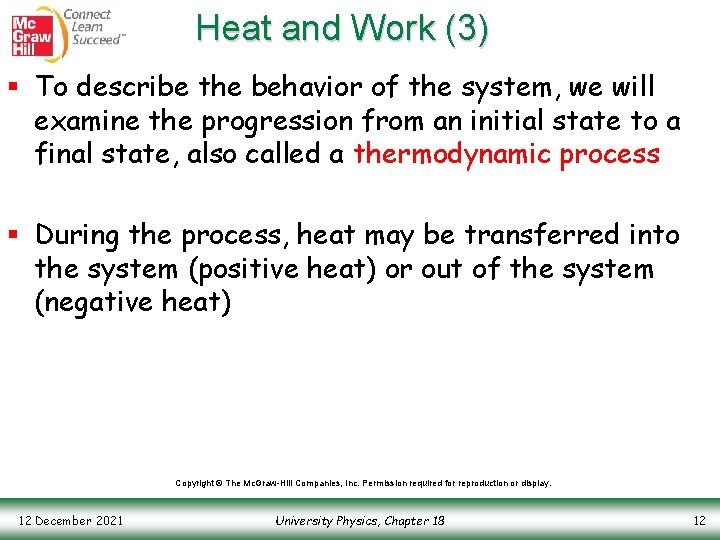
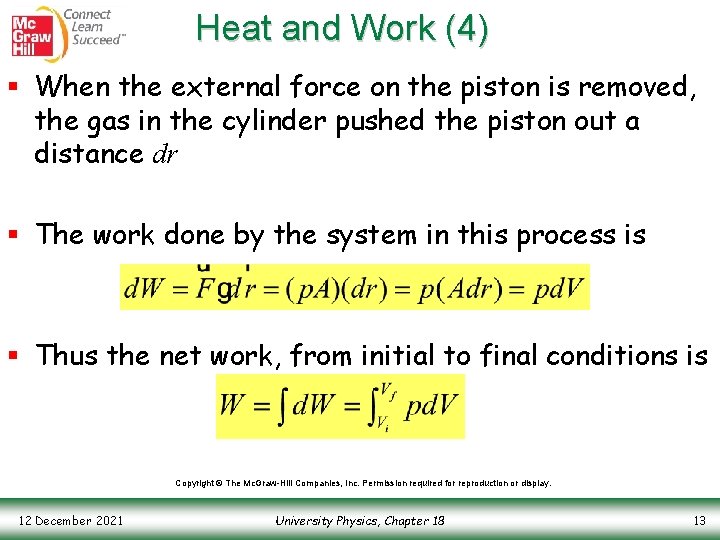
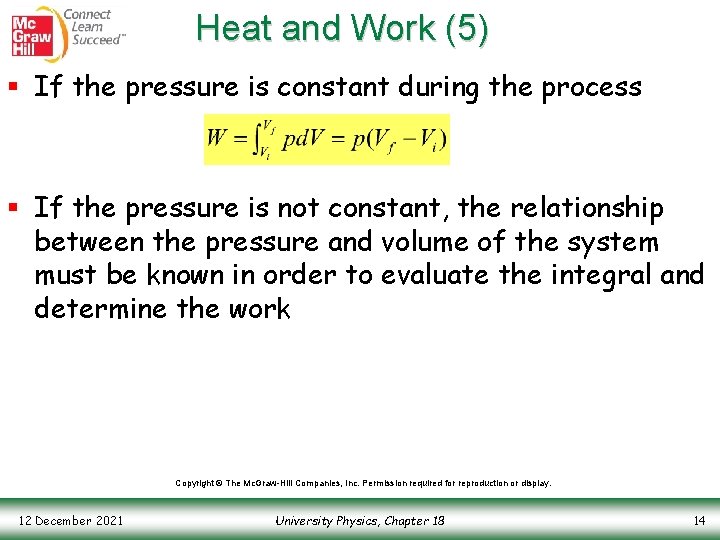
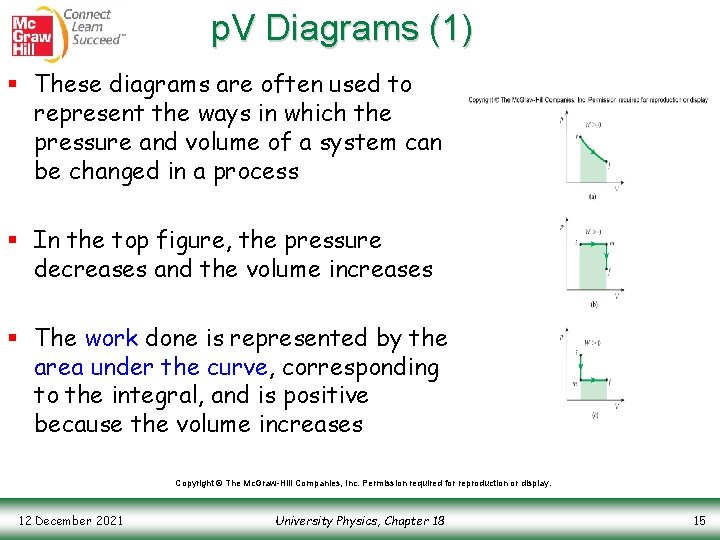
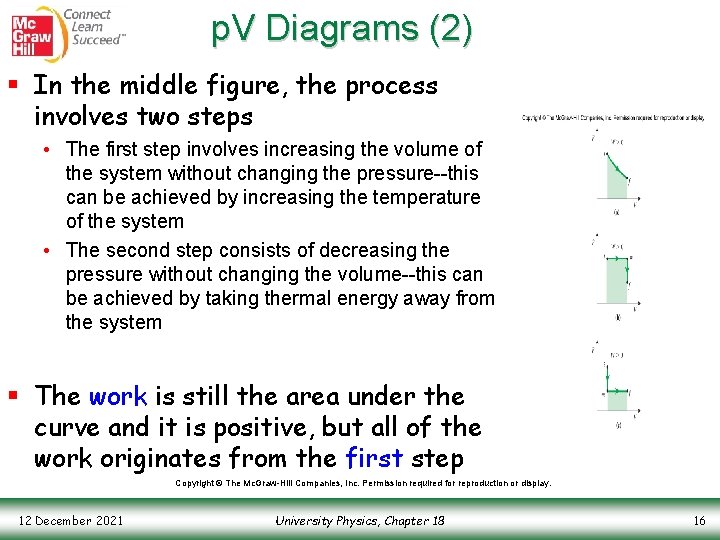
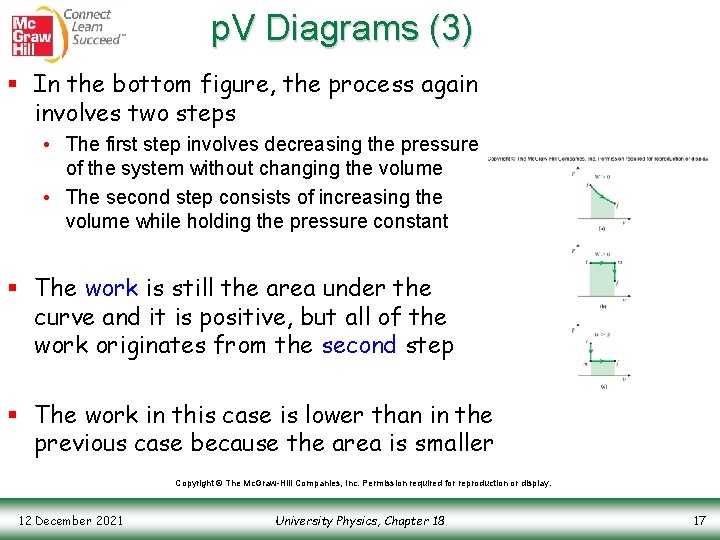
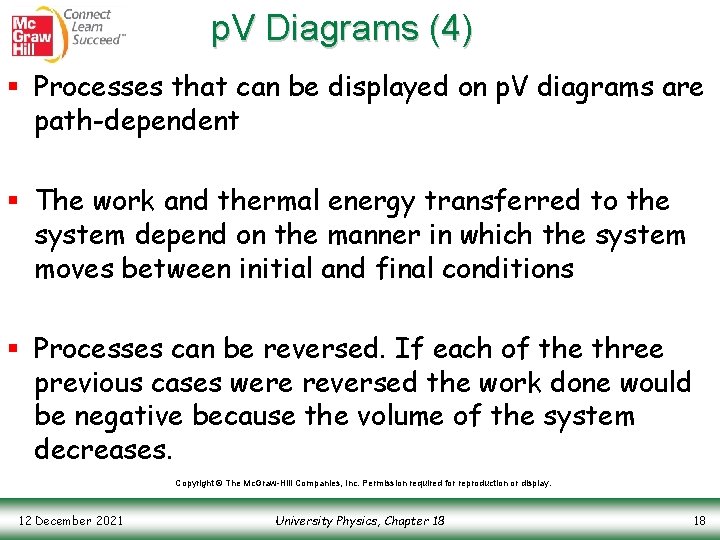
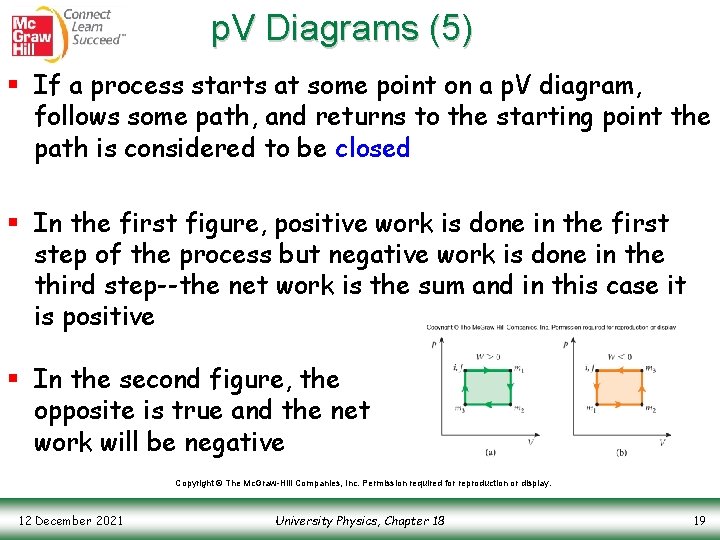
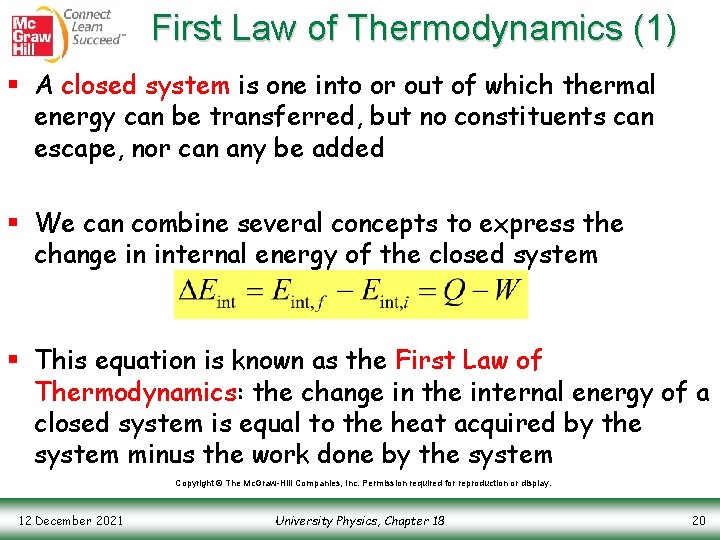
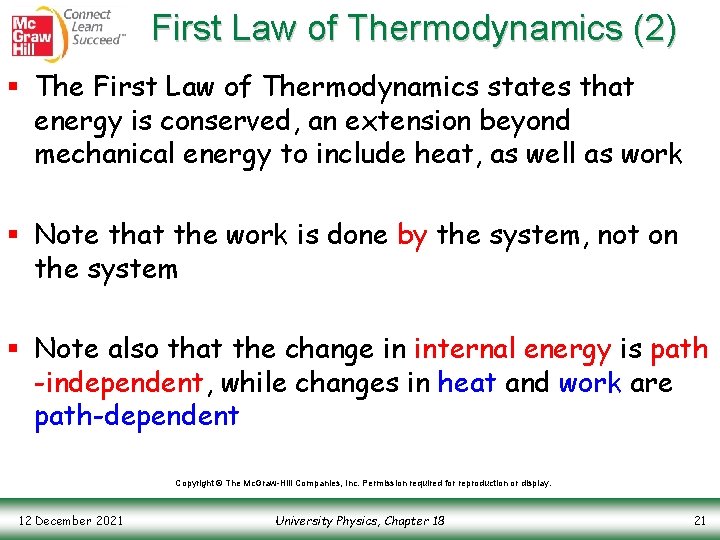
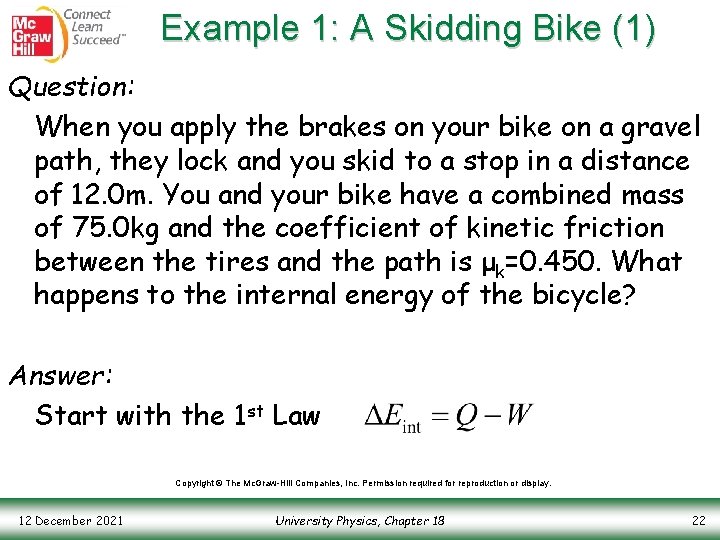
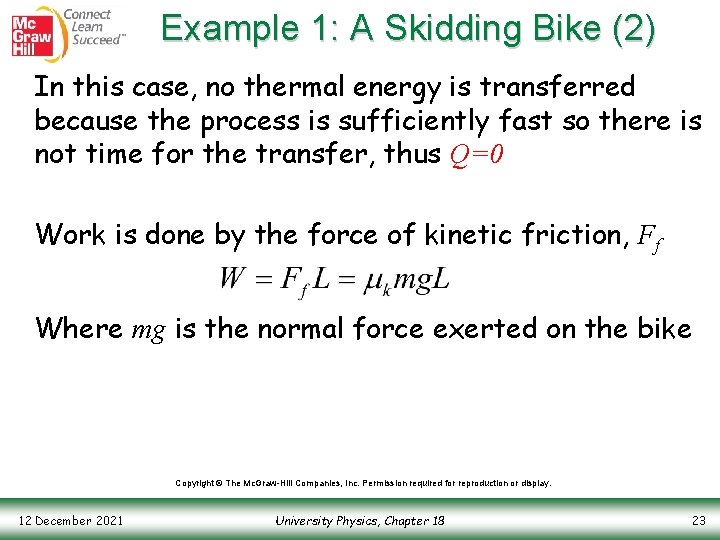
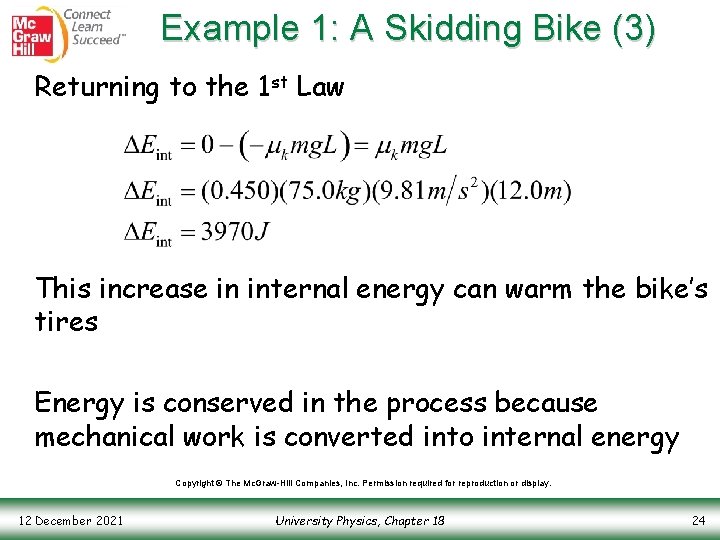
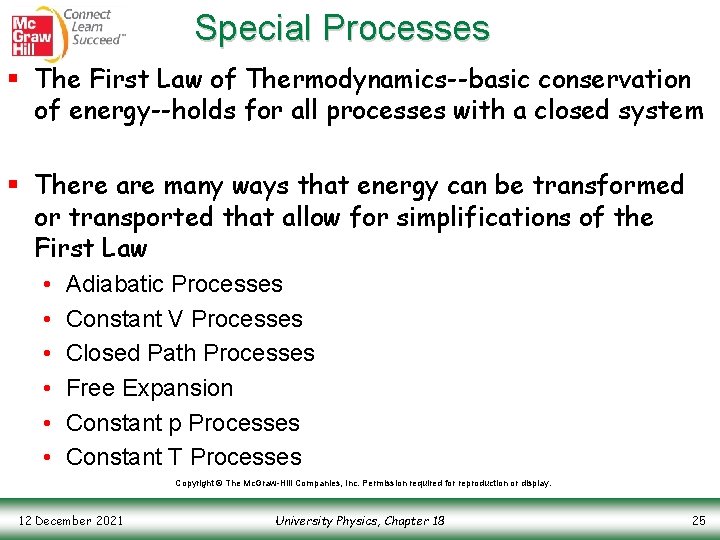
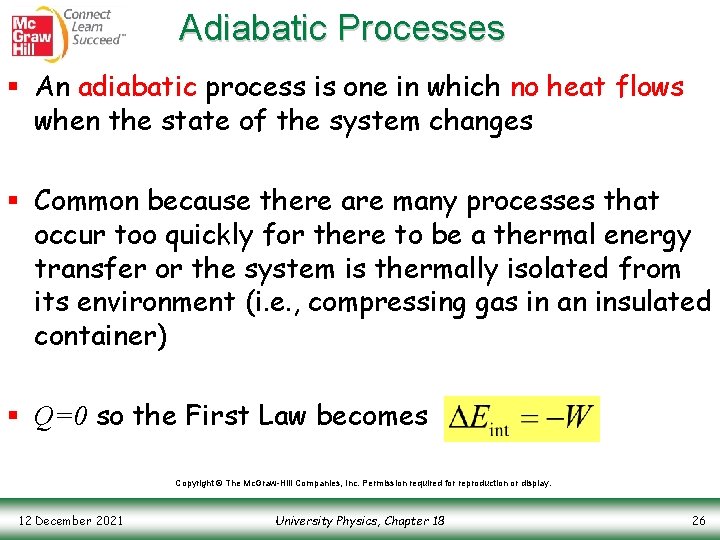
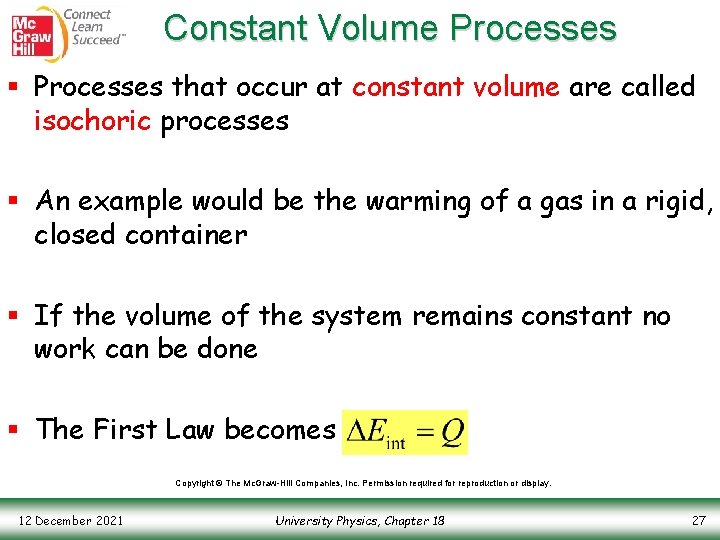
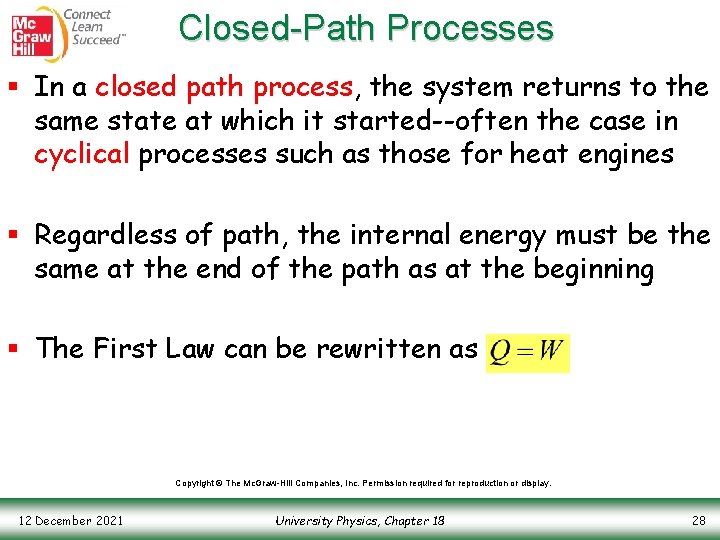
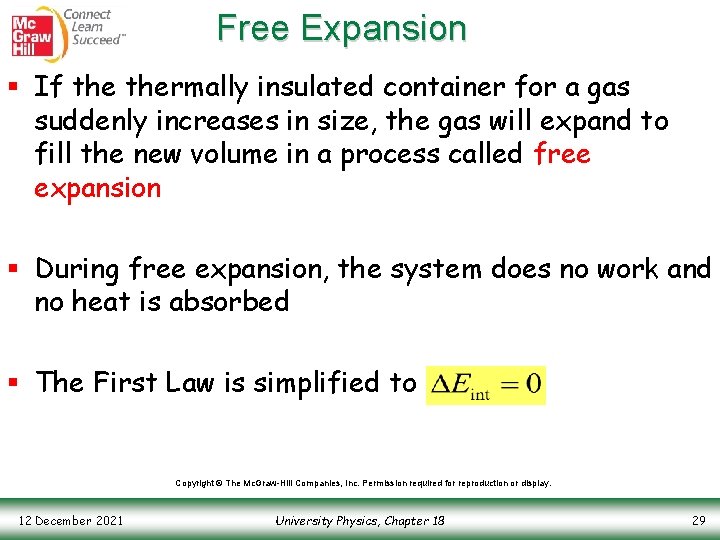
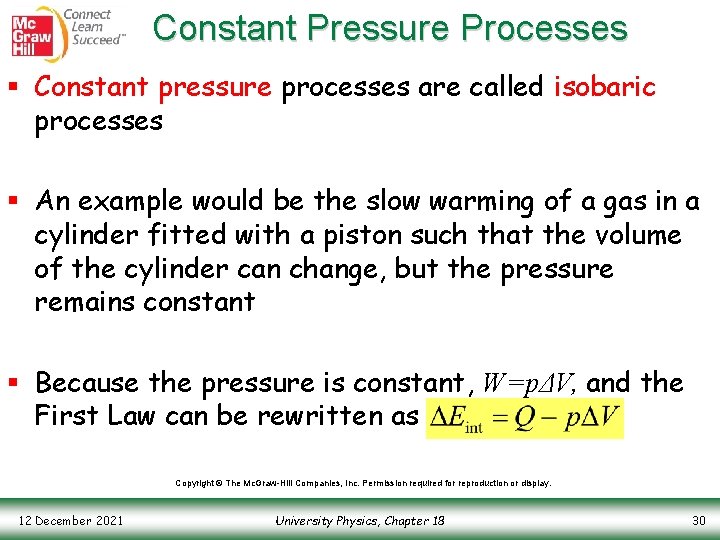
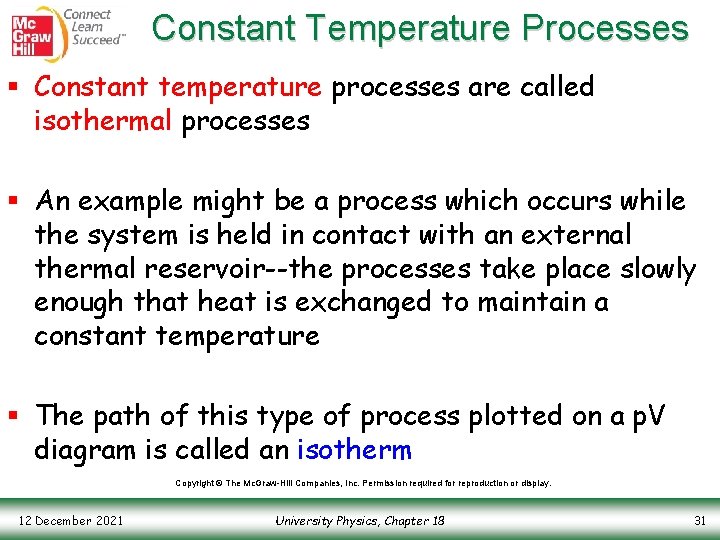
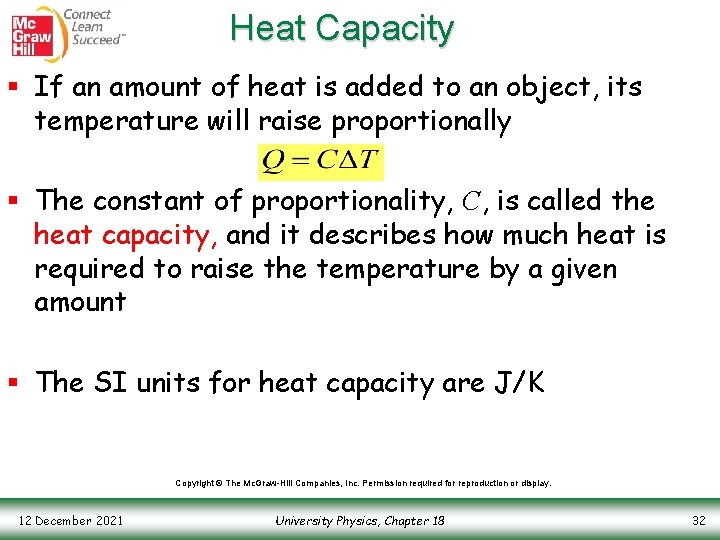
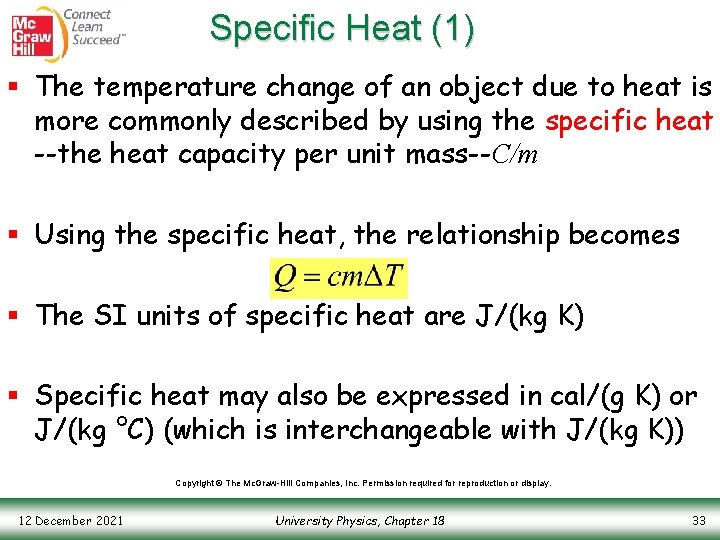
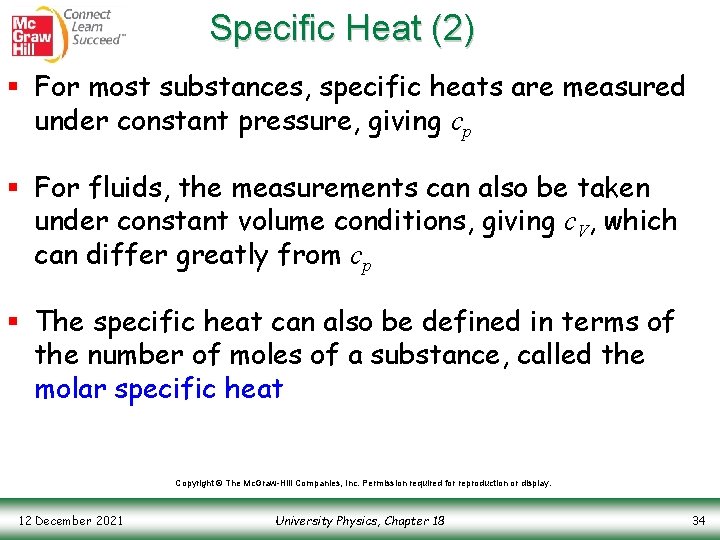
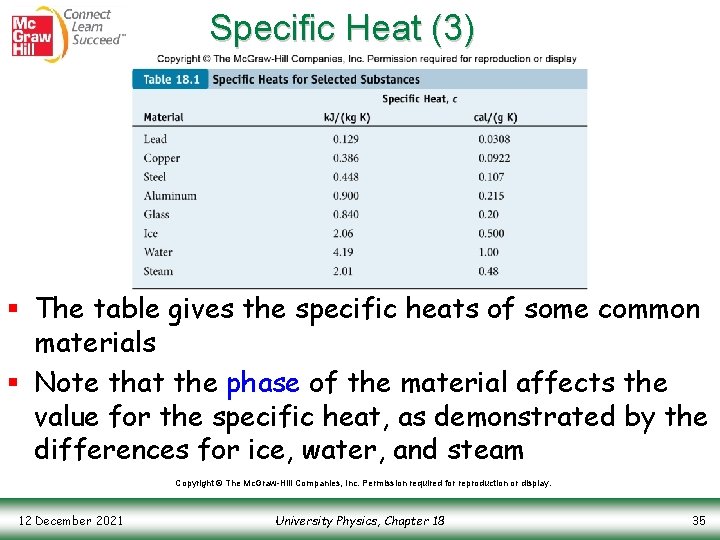
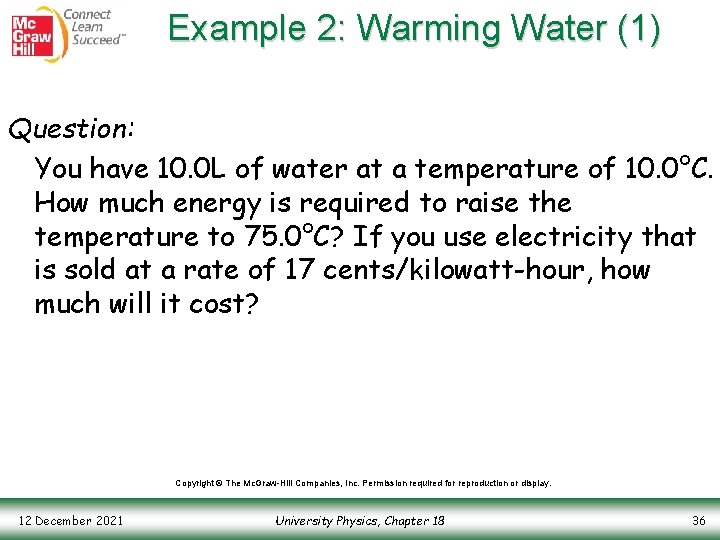
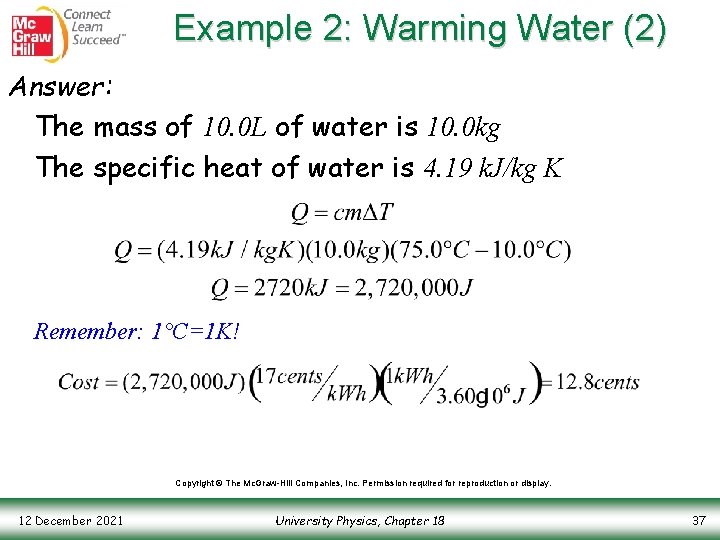
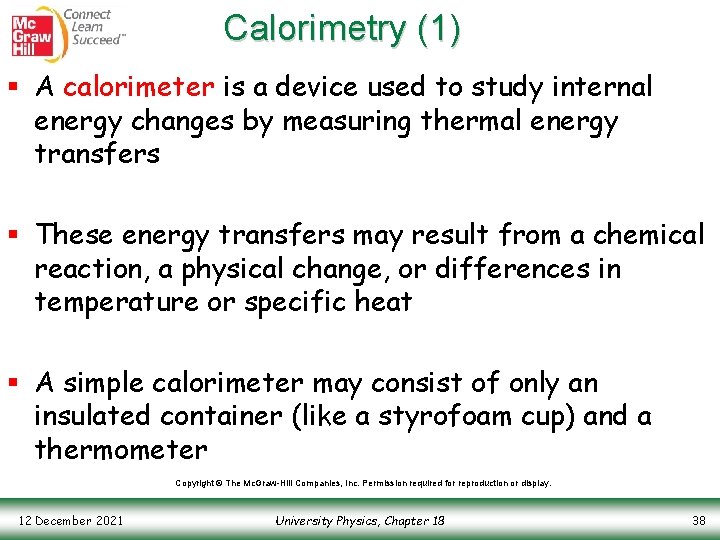
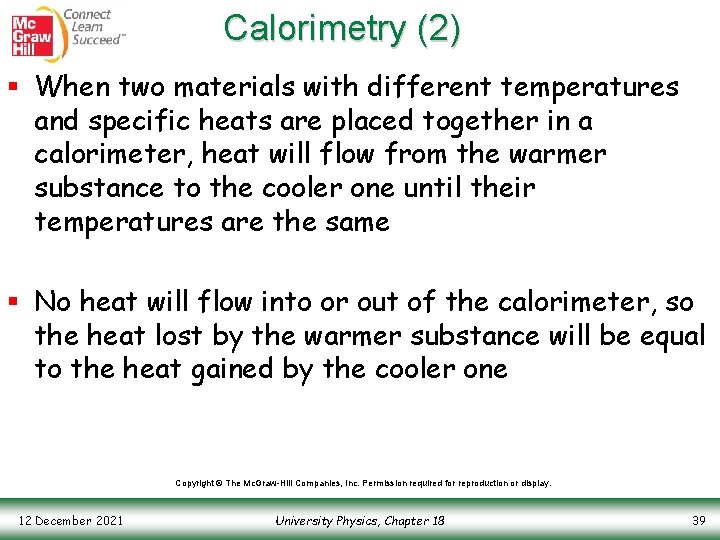
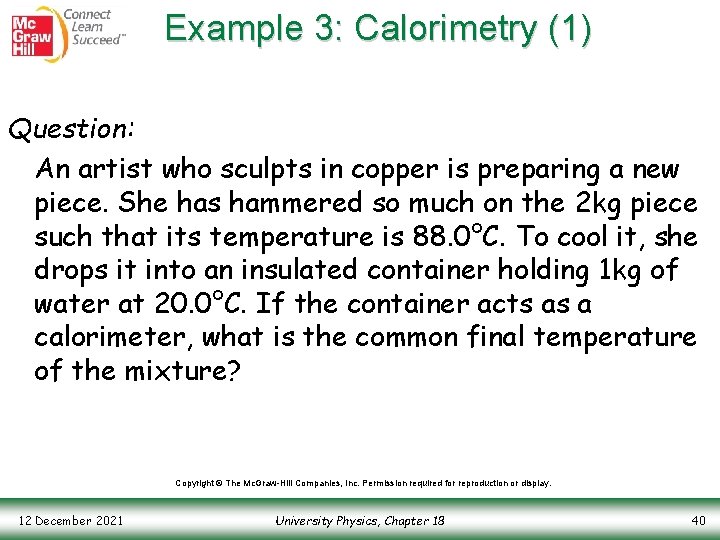
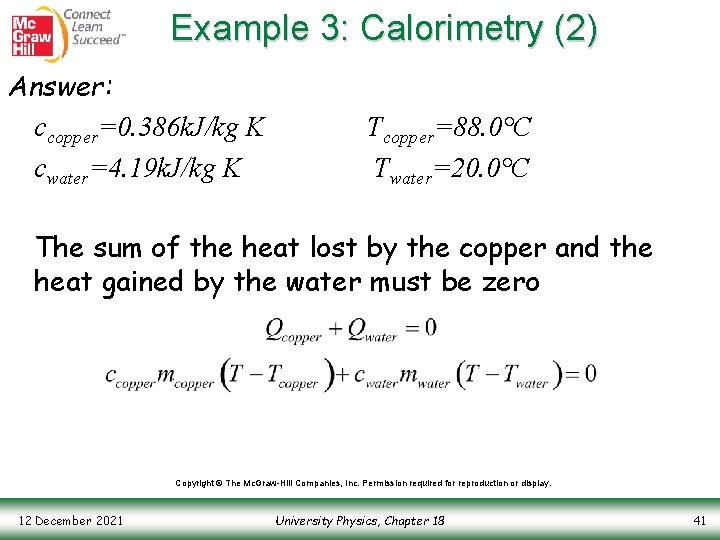
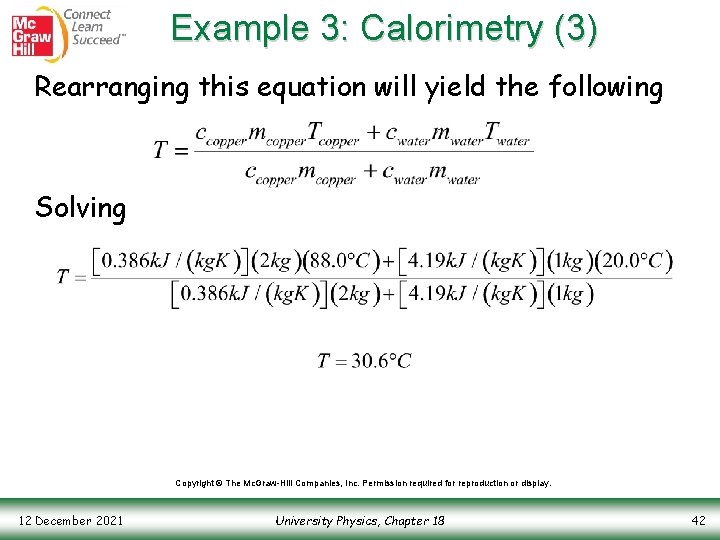
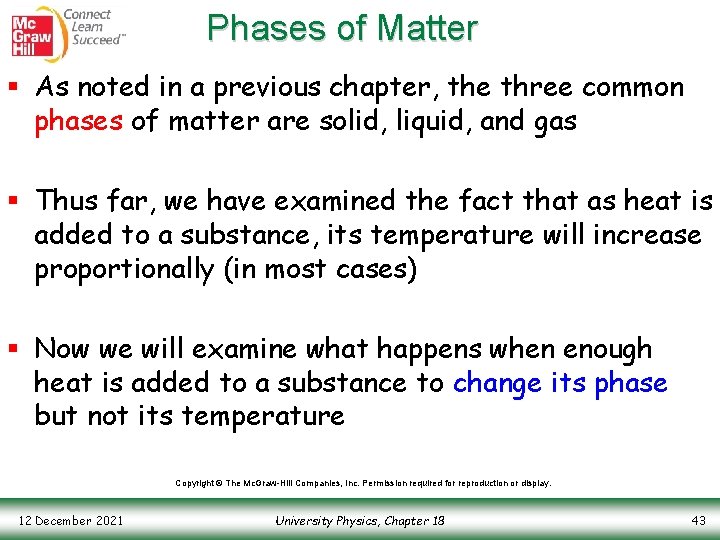
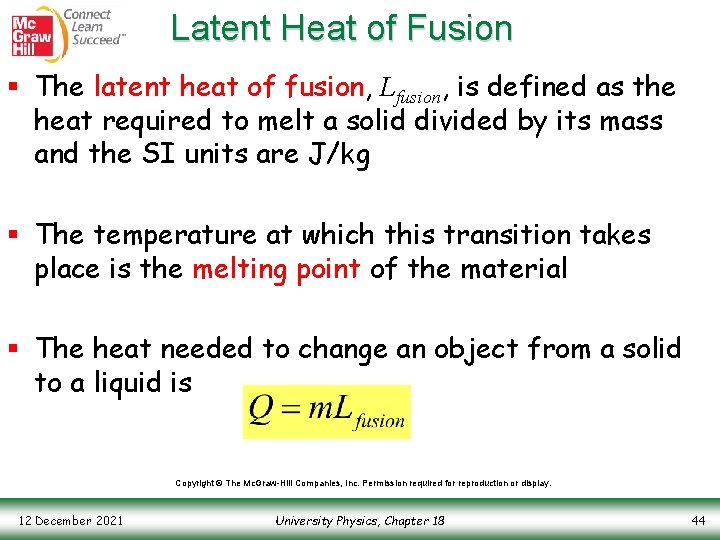
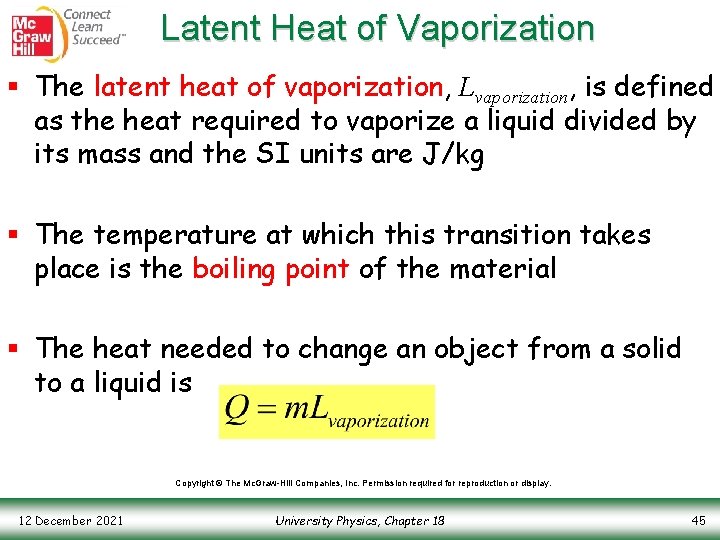
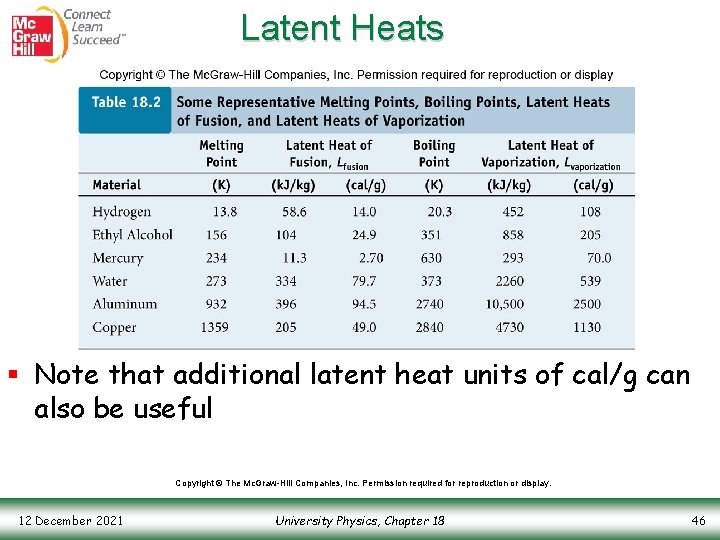
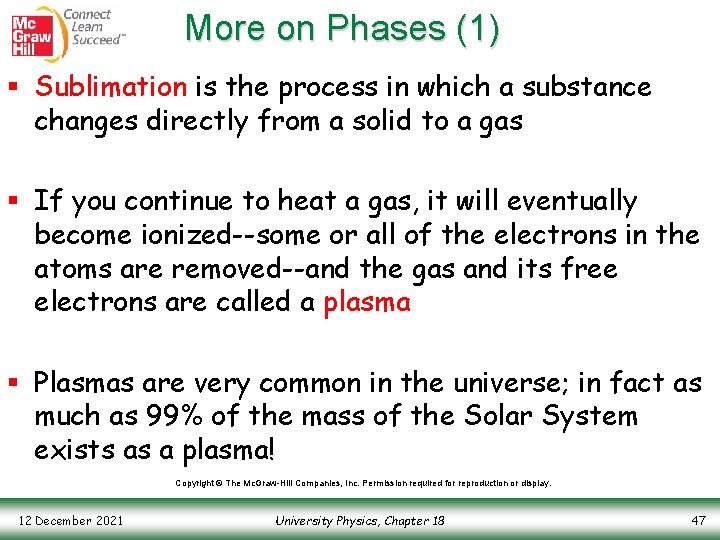
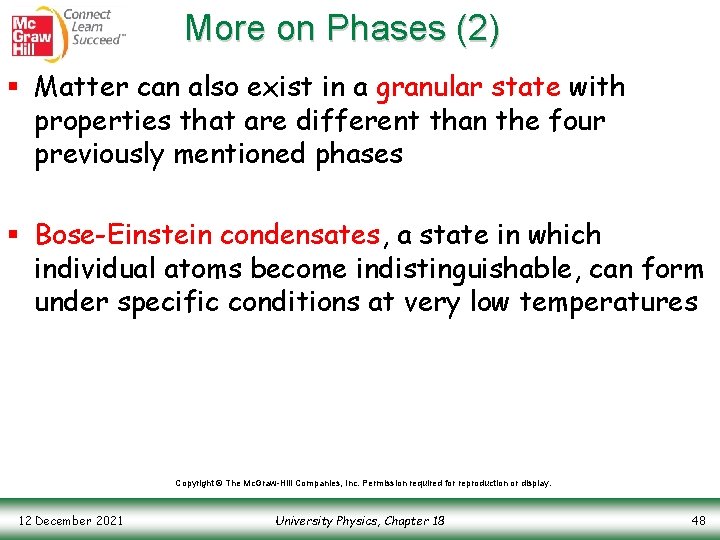
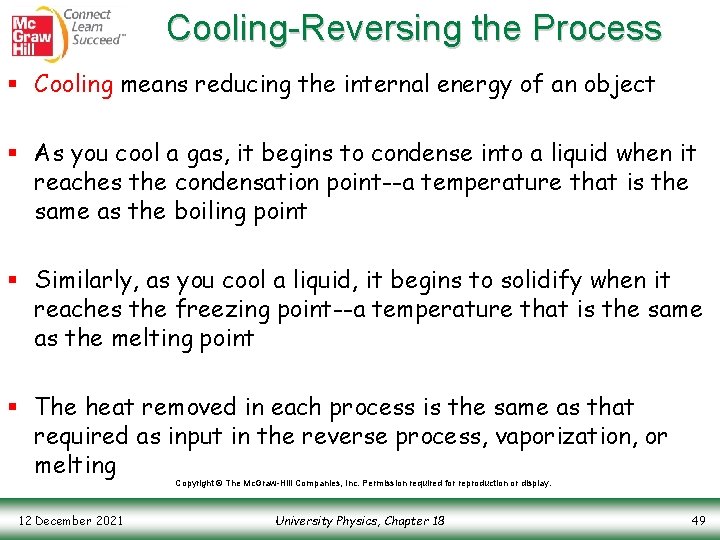
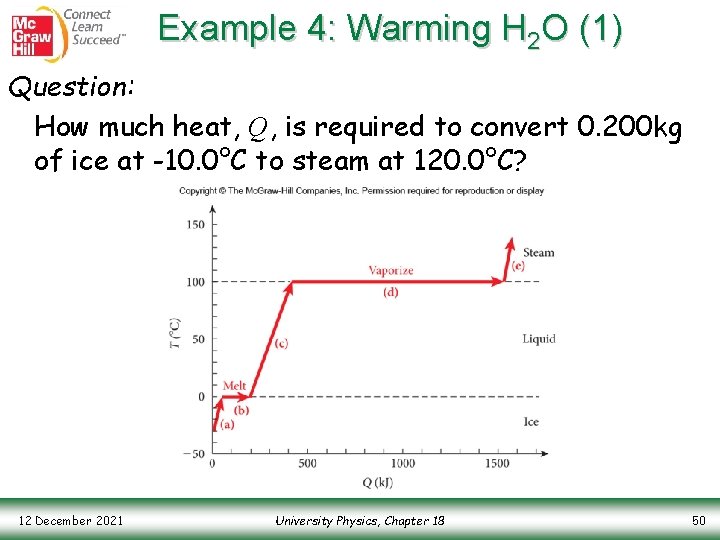
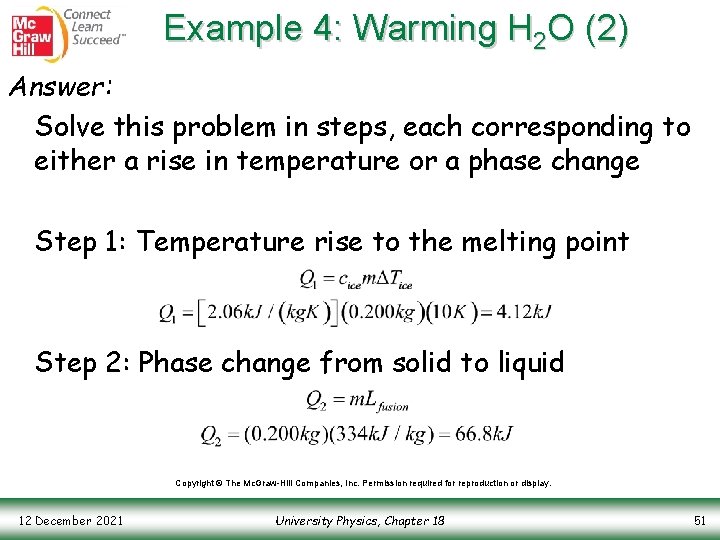
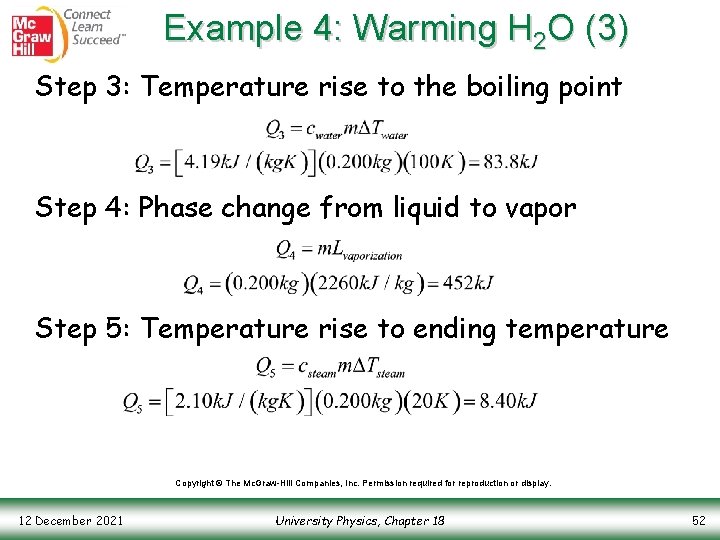
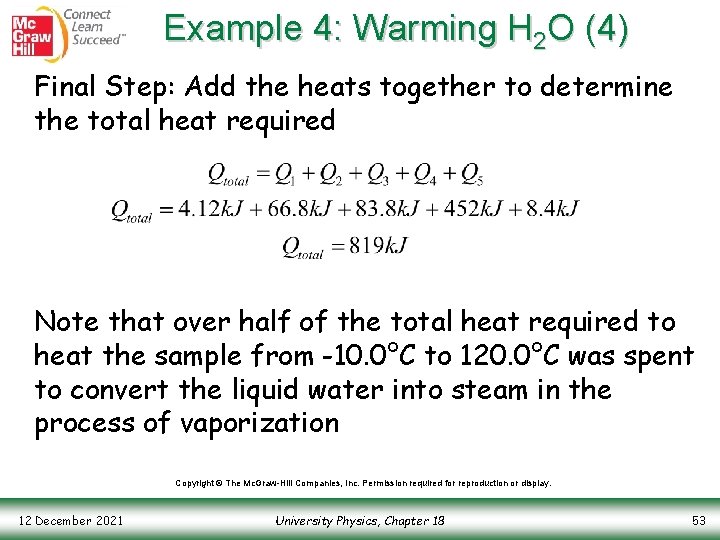
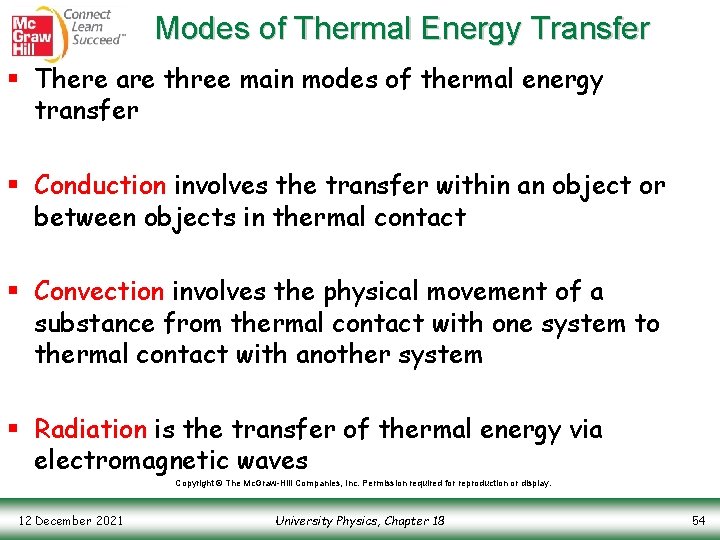
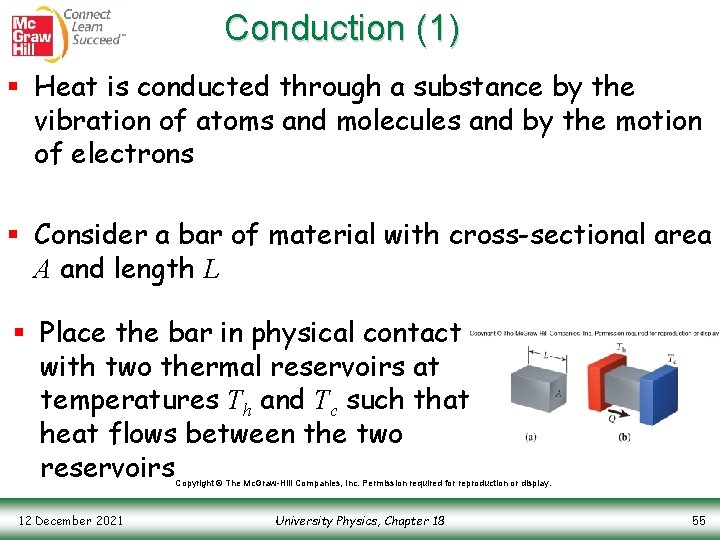
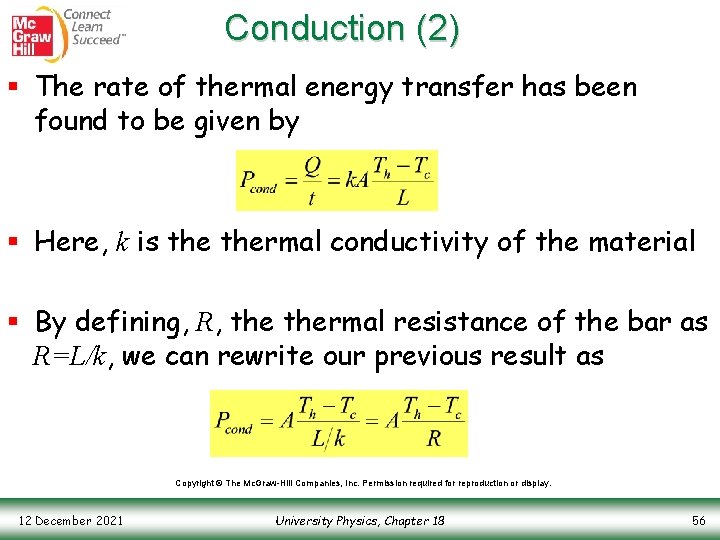
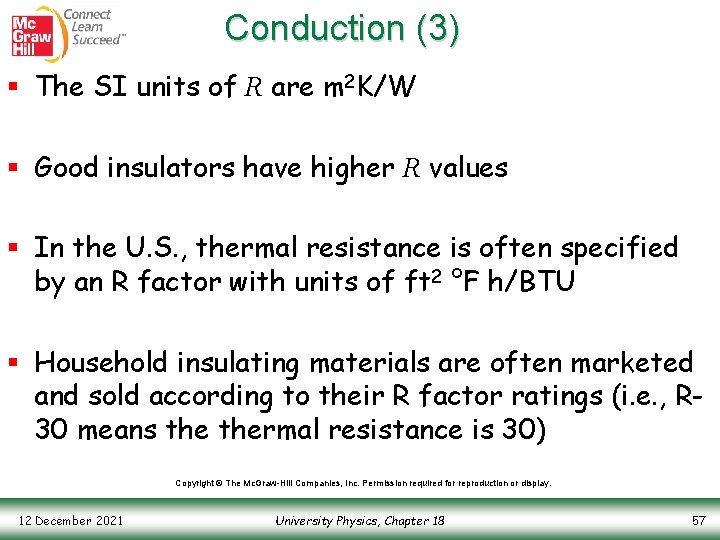
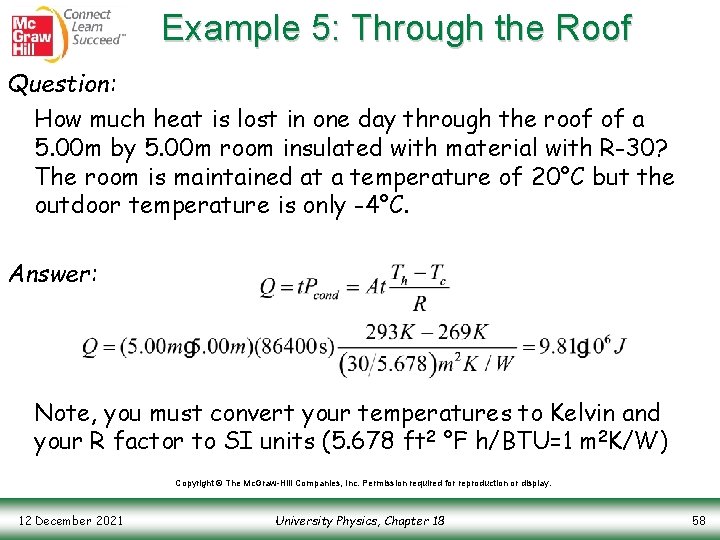
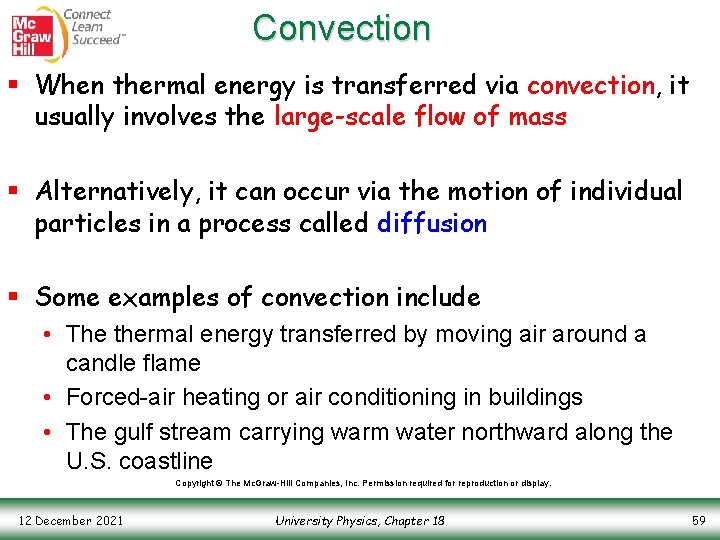
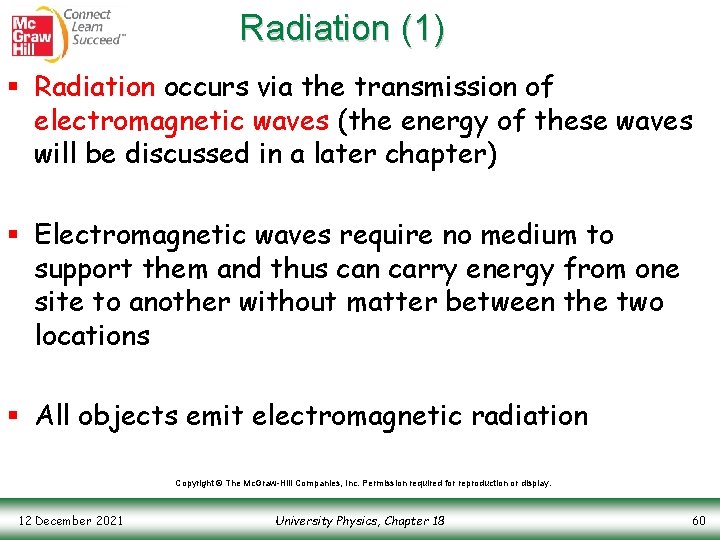
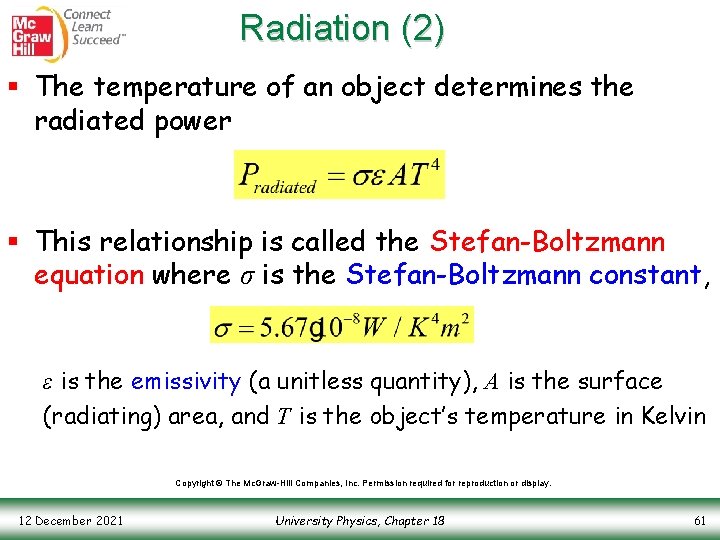
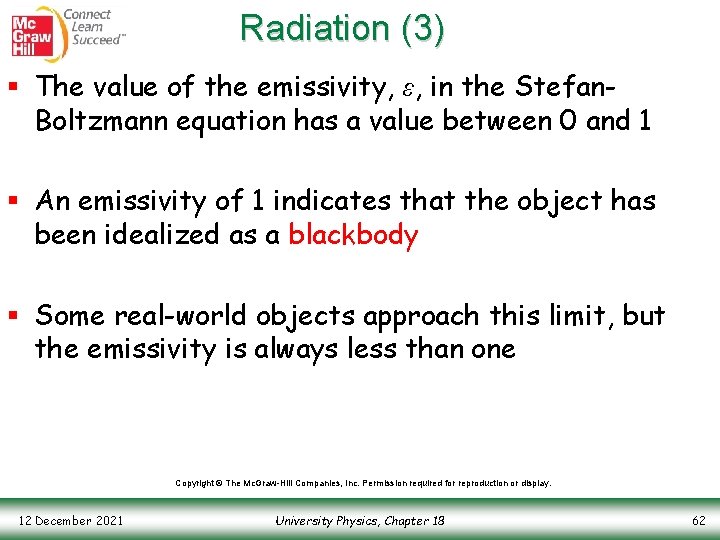
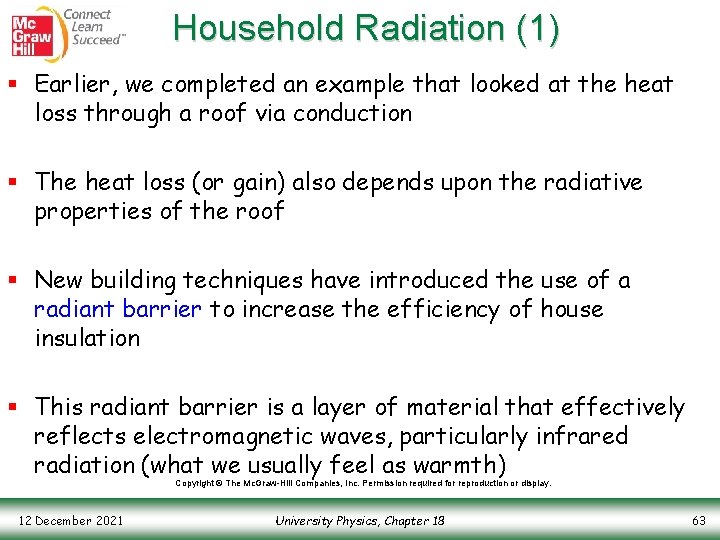
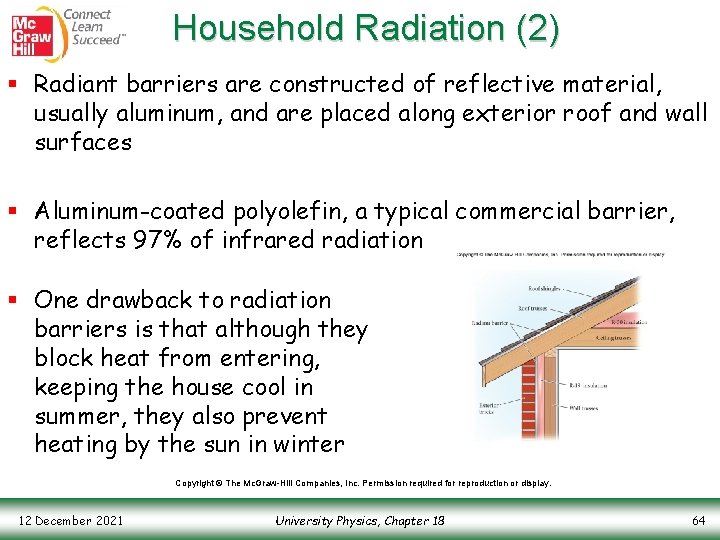
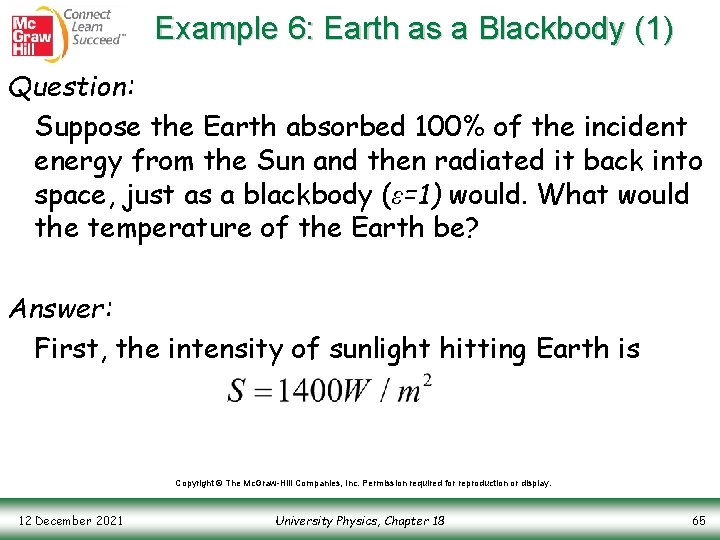
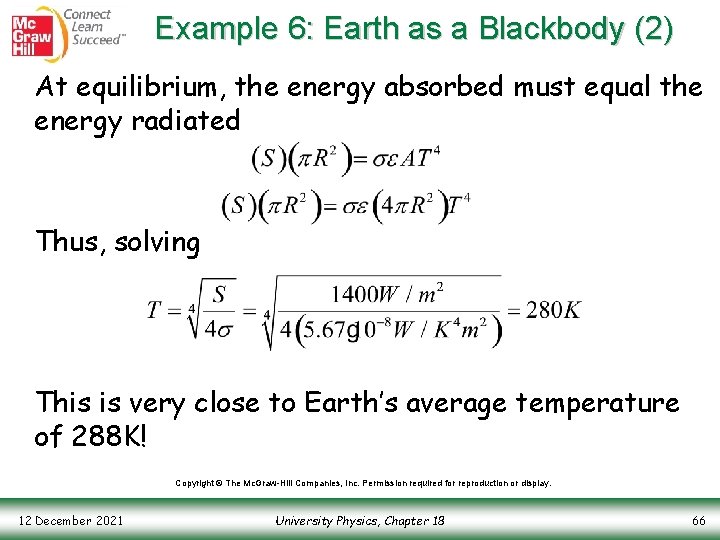
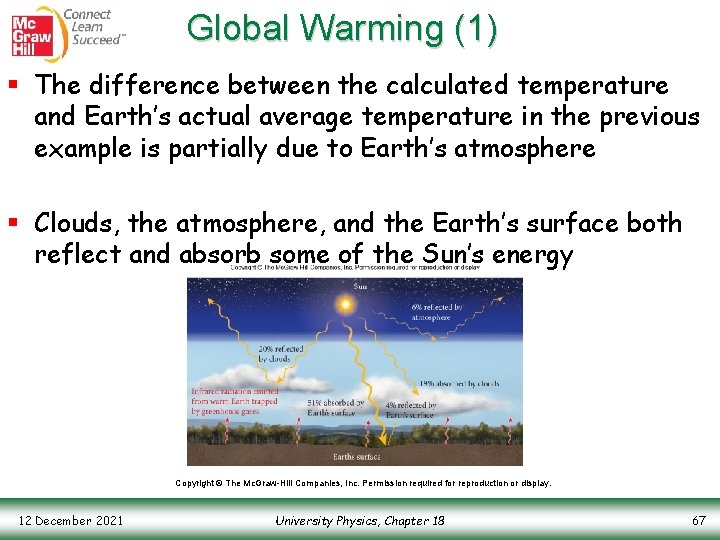
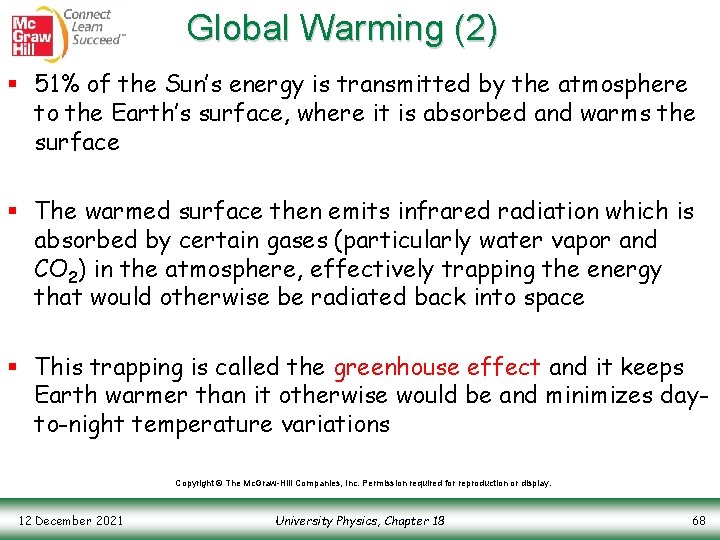
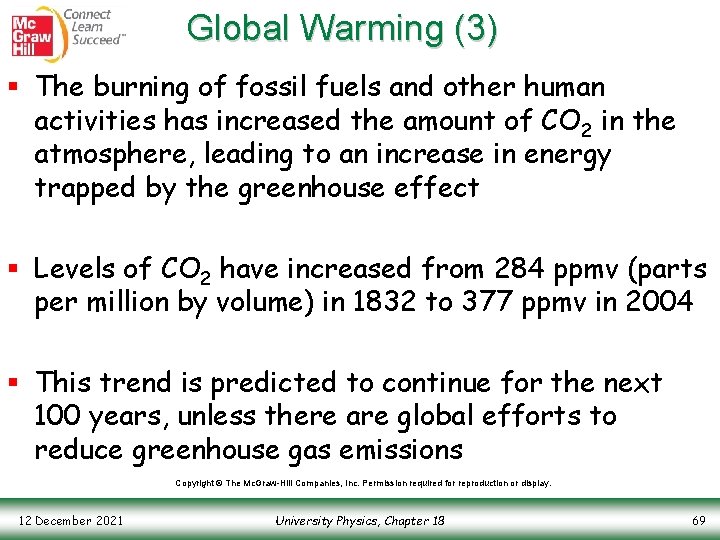
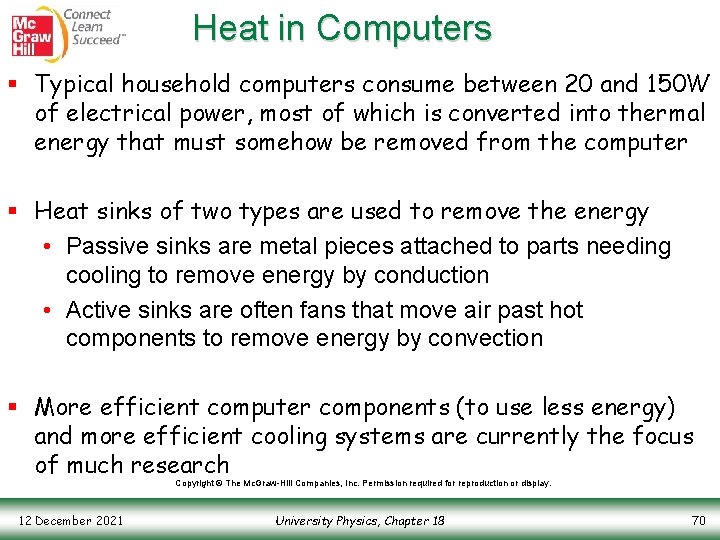
- Slides: 70
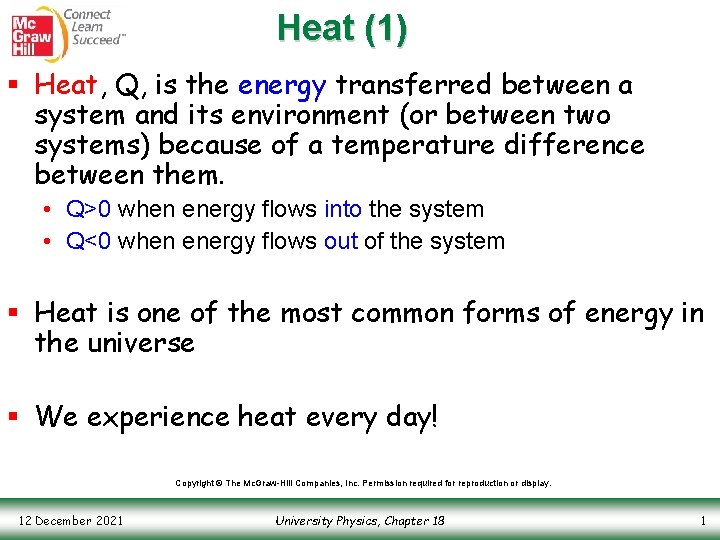
Heat (1) § Heat, Q, is the energy transferred between a system and its environment (or between two systems) because of a temperature difference between them. • Q>0 when energy flows into the system • Q<0 when energy flows out of the system § Heat is one of the most common forms of energy in the universe § We experience heat every day! Copyright © The Mc. Graw-Hill Companies, Inc. Permission required for reproduction or display. 12 December 2021 University Physics, Chapter 18 1
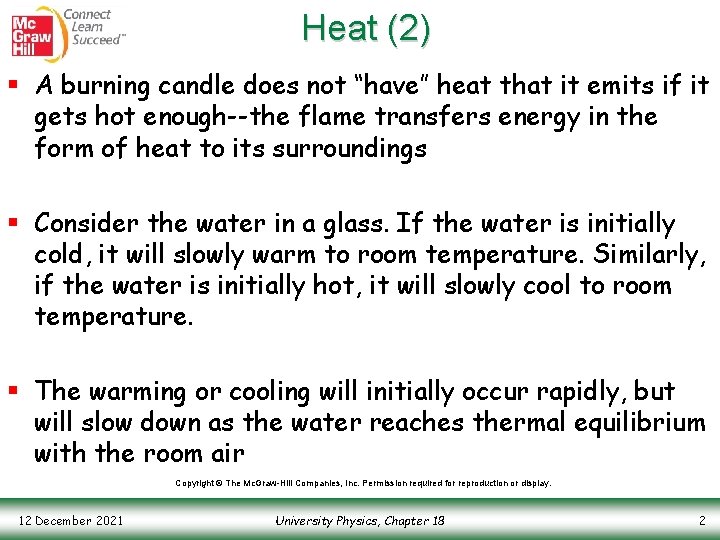
Heat (2) § A burning candle does not “have” heat that it emits if it gets hot enough--the flame transfers energy in the form of heat to its surroundings § Consider the water in a glass. If the water is initially cold, it will slowly warm to room temperature. Similarly, if the water is initially hot, it will slowly cool to room temperature. § The warming or cooling will initially occur rapidly, but will slow down as the water reaches thermal equilibrium with the room air Copyright © The Mc. Graw-Hill Companies, Inc. Permission required for reproduction or display. 12 December 2021 University Physics, Chapter 18 2
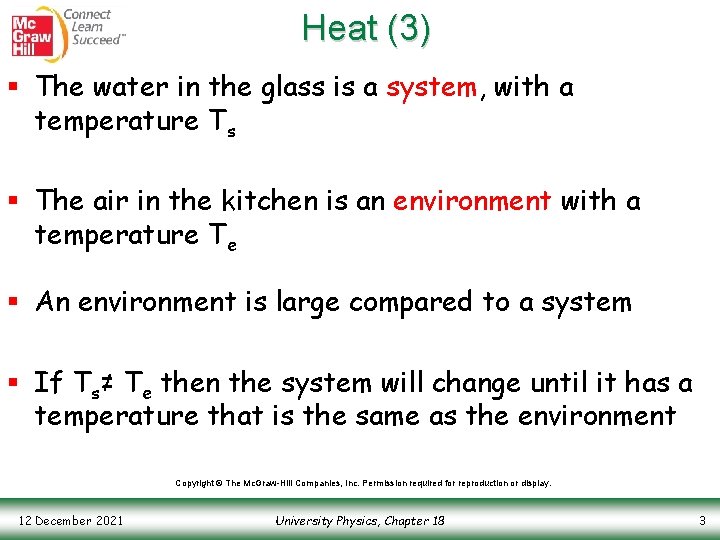
Heat (3) § The water in the glass is a system, with a temperature Ts § The air in the kitchen is an environment with a temperature Te § An environment is large compared to a system § If Ts≠ Te then the system will change until it has a temperature that is the same as the environment Copyright © The Mc. Graw-Hill Companies, Inc. Permission required for reproduction or display. 12 December 2021 University Physics, Chapter 18 3
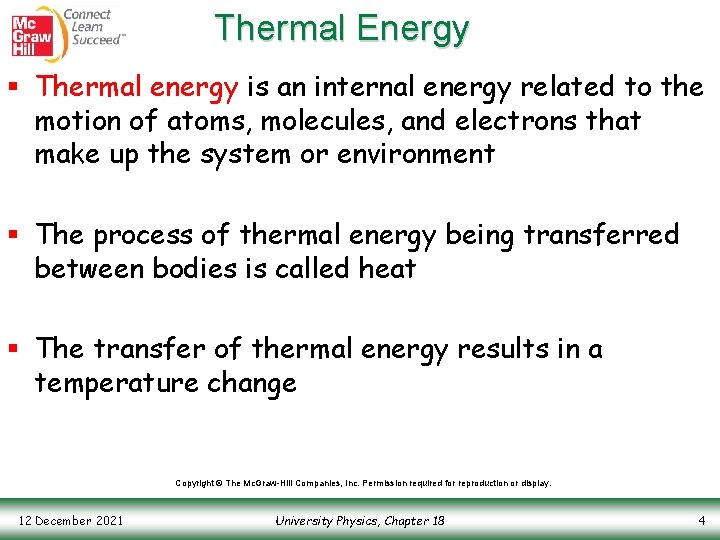
Thermal Energy § Thermal energy is an internal energy related to the motion of atoms, molecules, and electrons that make up the system or environment § The process of thermal energy being transferred between bodies is called heat § The transfer of thermal energy results in a temperature change Copyright © The Mc. Graw-Hill Companies, Inc. Permission required for reproduction or display. 12 December 2021 University Physics, Chapter 18 4
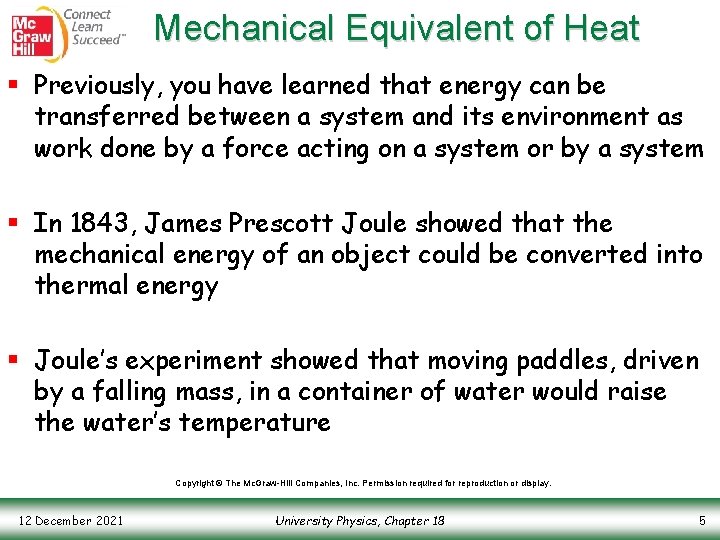
Mechanical Equivalent of Heat § Previously, you have learned that energy can be transferred between a system and its environment as work done by a force acting on a system or by a system § In 1843, James Prescott Joule showed that the mechanical energy of an object could be converted into thermal energy § Joule’s experiment showed that moving paddles, driven by a falling mass, in a container of water would raise the water’s temperature Copyright © The Mc. Graw-Hill Companies, Inc. Permission required for reproduction or display. 12 December 2021 University Physics, Chapter 18 5
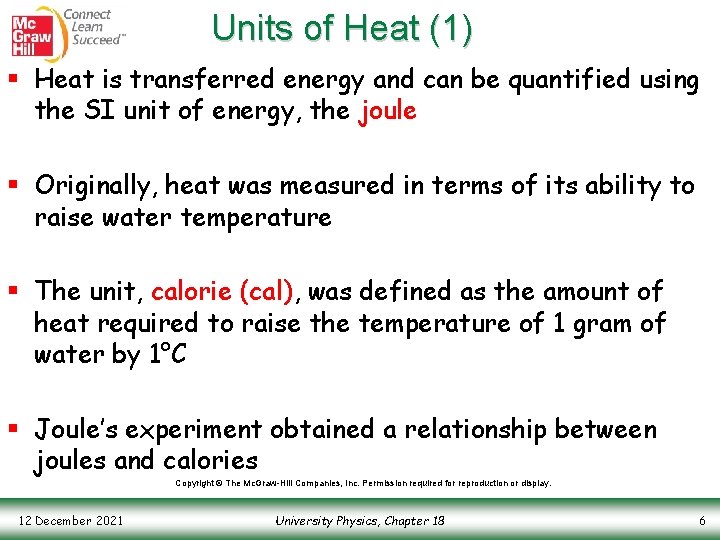
Units of Heat (1) § Heat is transferred energy and can be quantified using the SI unit of energy, the joule § Originally, heat was measured in terms of its ability to raise water temperature § The unit, calorie (cal), was defined as the amount of heat required to raise the temperature of 1 gram of water by 1°C § Joule’s experiment obtained a relationship between joules and calories Copyright © The Mc. Graw-Hill Companies, Inc. Permission required for reproduction or display. 12 December 2021 University Physics, Chapter 18 6
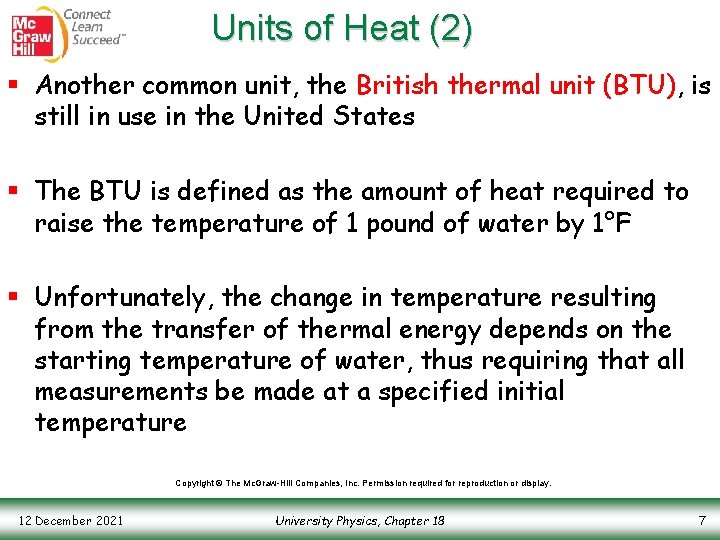
Units of Heat (2) § Another common unit, the British thermal unit (BTU), is still in use in the United States § The BTU is defined as the amount of heat required to raise the temperature of 1 pound of water by 1°F § Unfortunately, the change in temperature resulting from the transfer of thermal energy depends on the starting temperature of water, thus requiring that all measurements be made at a specified initial temperature Copyright © The Mc. Graw-Hill Companies, Inc. Permission required for reproduction or display. 12 December 2021 University Physics, Chapter 18 7
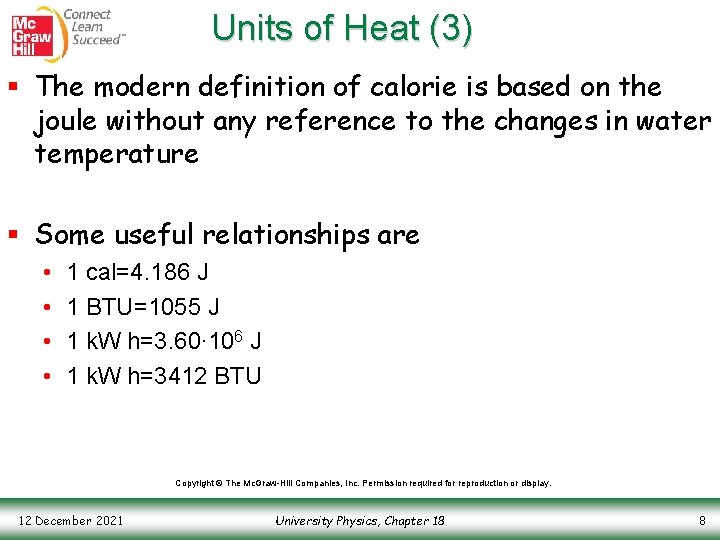
Units of Heat (3) § The modern definition of calorie is based on the joule without any reference to the changes in water temperature § Some useful relationships are • • 1 cal=4. 186 J 1 BTU=1055 J 1 k. W h=3. 60∙ 106 J 1 k. W h=3412 BTU Copyright © The Mc. Graw-Hill Companies, Inc. Permission required for reproduction or display. 12 December 2021 University Physics, Chapter 18 8
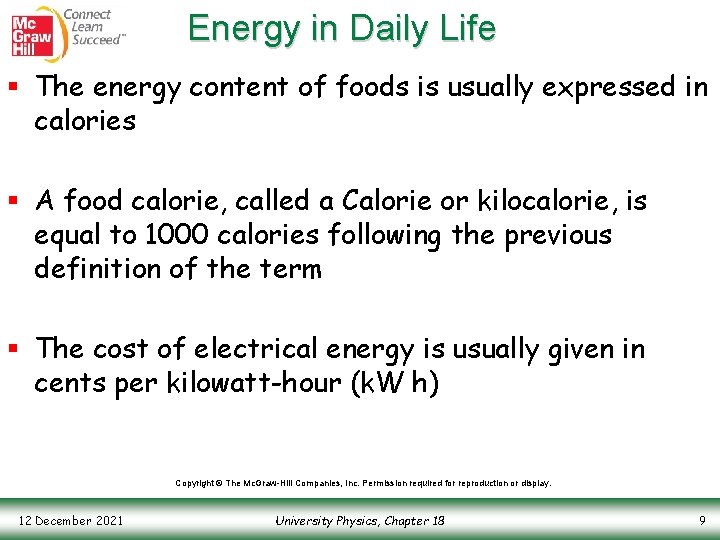
Energy in Daily Life § The energy content of foods is usually expressed in calories § A food calorie, called a Calorie or kilocalorie, is equal to 1000 calories following the previous definition of the term § The cost of electrical energy is usually given in cents per kilowatt-hour (k. W h) Copyright © The Mc. Graw-Hill Companies, Inc. Permission required for reproduction or display. 12 December 2021 University Physics, Chapter 18 9
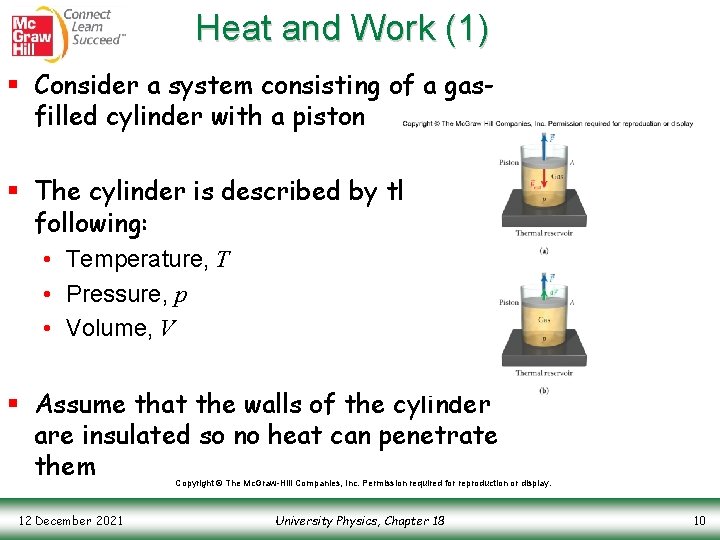
Heat and Work (1) § Consider a system consisting of a gasfilled cylinder with a piston § The cylinder is described by the following: • Temperature, T • Pressure, p • Volume, V § Assume that the walls of the cylinder are insulated so no heat can penetrate them Copyright © The Mc. Graw-Hill Companies, Inc. Permission required for reproduction or display. 12 December 2021 University Physics, Chapter 18 10
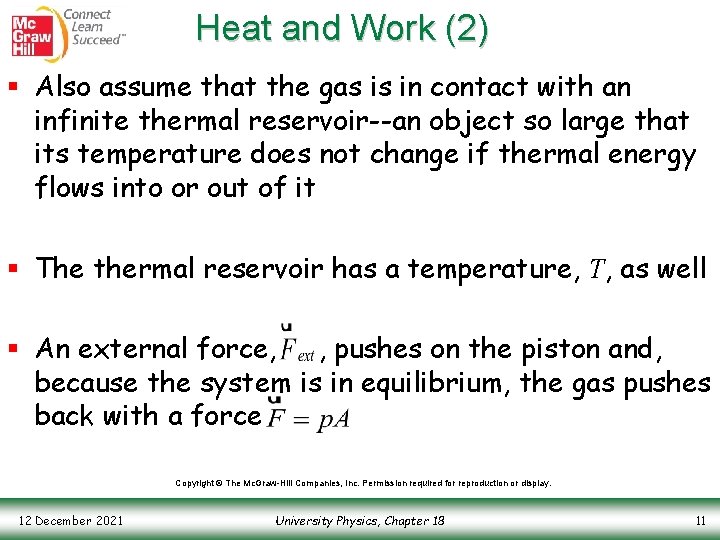
Heat and Work (2) § Also assume that the gas is in contact with an infinite thermal reservoir--an object so large that its temperature does not change if thermal energy flows into or out of it § The thermal reservoir has a temperature, T, as well § An external force, , pushes on the piston and, because the system is in equilibrium, the gas pushes back with a force Copyright © The Mc. Graw-Hill Companies, Inc. Permission required for reproduction or display. 12 December 2021 University Physics, Chapter 18 11
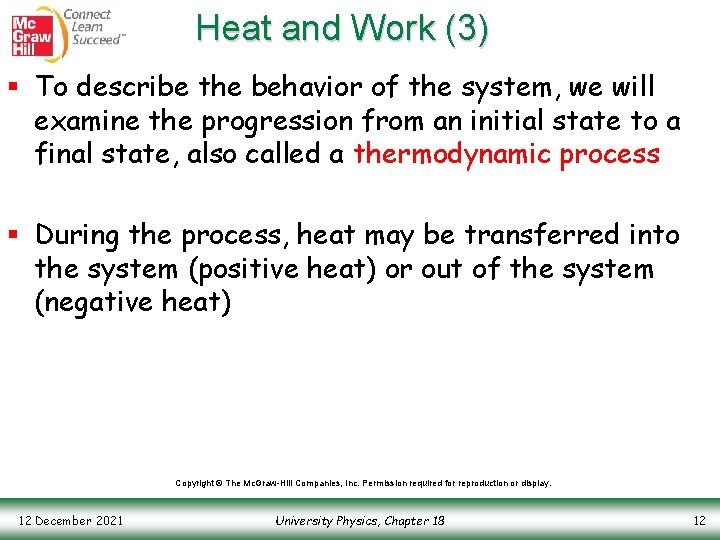
Heat and Work (3) § To describe the behavior of the system, we will examine the progression from an initial state to a final state, also called a thermodynamic process § During the process, heat may be transferred into the system (positive heat) or out of the system (negative heat) Copyright © The Mc. Graw-Hill Companies, Inc. Permission required for reproduction or display. 12 December 2021 University Physics, Chapter 18 12
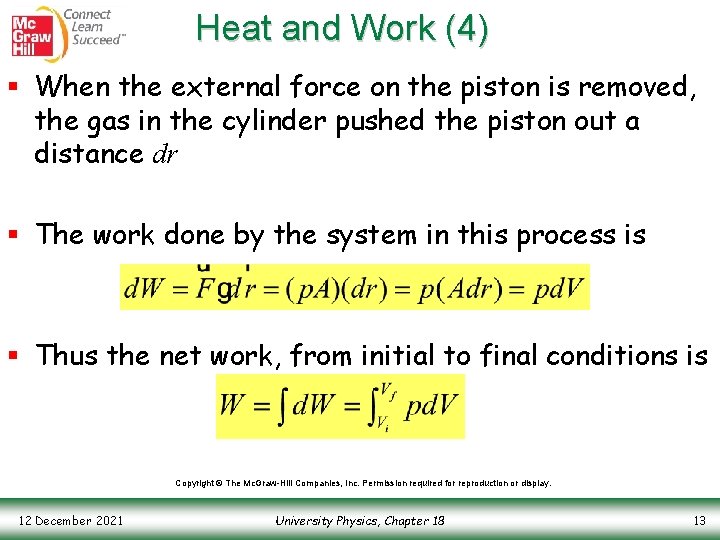
Heat and Work (4) § When the external force on the piston is removed, the gas in the cylinder pushed the piston out a distance dr § The work done by the system in this process is § Thus the net work, from initial to final conditions is Copyright © The Mc. Graw-Hill Companies, Inc. Permission required for reproduction or display. 12 December 2021 University Physics, Chapter 18 13
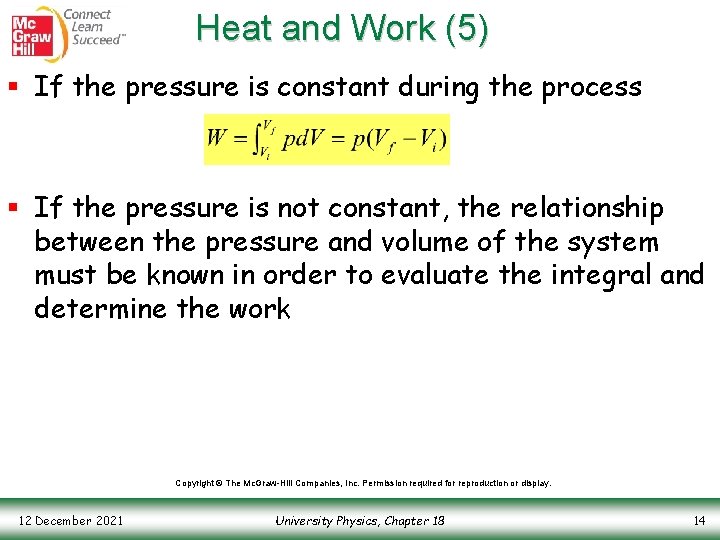
Heat and Work (5) § If the pressure is constant during the process § If the pressure is not constant, the relationship between the pressure and volume of the system must be known in order to evaluate the integral and determine the work Copyright © The Mc. Graw-Hill Companies, Inc. Permission required for reproduction or display. 12 December 2021 University Physics, Chapter 18 14
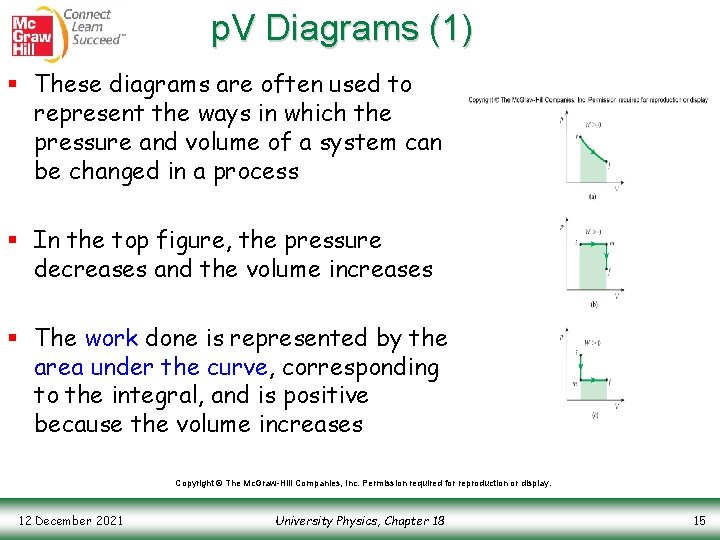
p. V Diagrams (1) § These diagrams are often used to represent the ways in which the pressure and volume of a system can be changed in a process § In the top figure, the pressure decreases and the volume increases § The work done is represented by the area under the curve, corresponding to the integral, and is positive because the volume increases Copyright © The Mc. Graw-Hill Companies, Inc. Permission required for reproduction or display. 12 December 2021 University Physics, Chapter 18 15
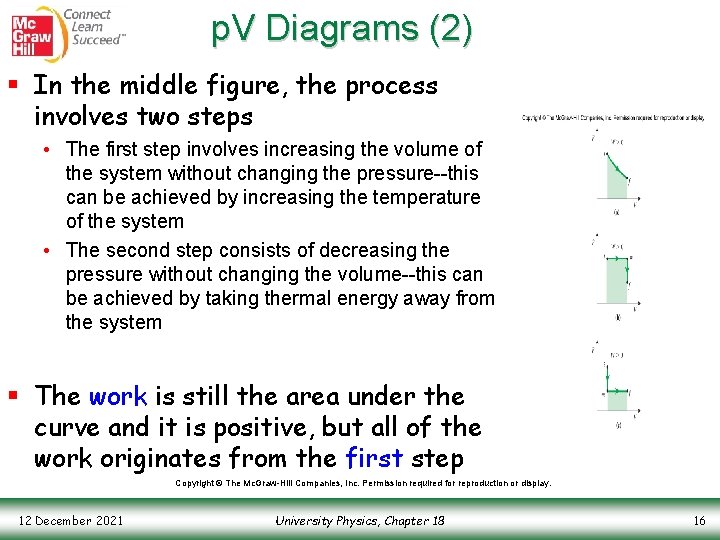
p. V Diagrams (2) § In the middle figure, the process involves two steps • The first step involves increasing the volume of the system without changing the pressure--this can be achieved by increasing the temperature of the system • The second step consists of decreasing the pressure without changing the volume--this can be achieved by taking thermal energy away from the system § The work is still the area under the curve and it is positive, but all of the work originates from the first step Copyright © The Mc. Graw-Hill Companies, Inc. Permission required for reproduction or display. 12 December 2021 University Physics, Chapter 18 16
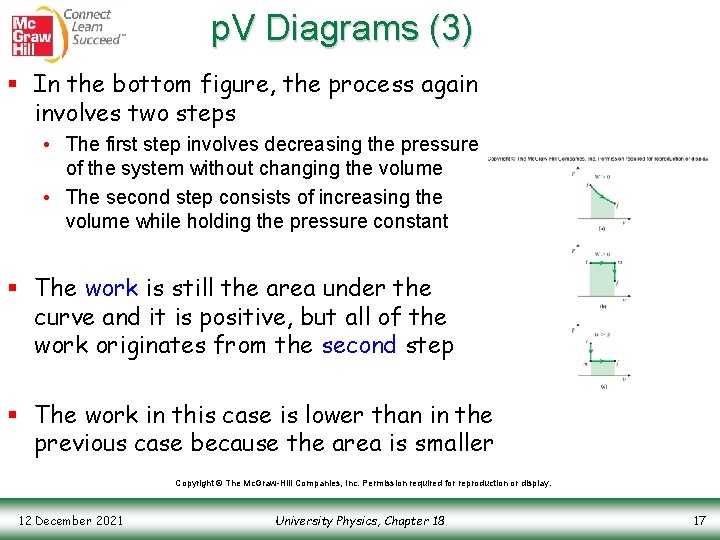
p. V Diagrams (3) § In the bottom figure, the process again involves two steps • The first step involves decreasing the pressure of the system without changing the volume • The second step consists of increasing the volume while holding the pressure constant § The work is still the area under the curve and it is positive, but all of the work originates from the second step § The work in this case is lower than in the previous case because the area is smaller Copyright © The Mc. Graw-Hill Companies, Inc. Permission required for reproduction or display. 12 December 2021 University Physics, Chapter 18 17
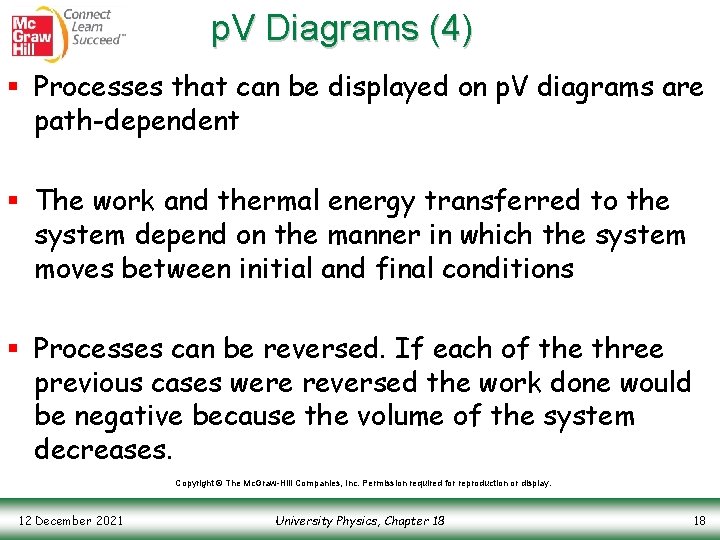
p. V Diagrams (4) § Processes that can be displayed on p. V diagrams are path-dependent § The work and thermal energy transferred to the system depend on the manner in which the system moves between initial and final conditions § Processes can be reversed. If each of the three previous cases were reversed the work done would be negative because the volume of the system decreases. Copyright © The Mc. Graw-Hill Companies, Inc. Permission required for reproduction or display. 12 December 2021 University Physics, Chapter 18 18
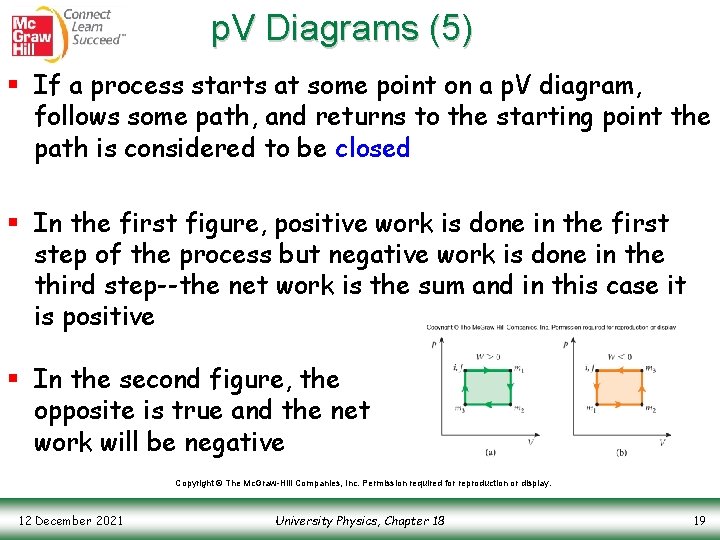
p. V Diagrams (5) § If a process starts at some point on a p. V diagram, follows some path, and returns to the starting point the path is considered to be closed § In the first figure, positive work is done in the first step of the process but negative work is done in the third step--the net work is the sum and in this case it is positive § In the second figure, the opposite is true and the net work will be negative Copyright © The Mc. Graw-Hill Companies, Inc. Permission required for reproduction or display. 12 December 2021 University Physics, Chapter 18 19
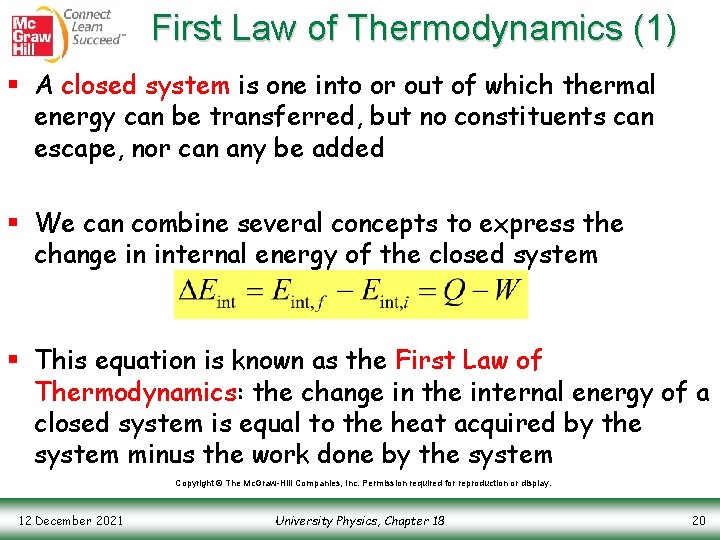
First Law of Thermodynamics (1) § A closed system is one into or out of which thermal energy can be transferred, but no constituents can escape, nor can any be added § We can combine several concepts to express the change in internal energy of the closed system § This equation is known as the First Law of Thermodynamics: the change in the internal energy of a closed system is equal to the heat acquired by the system minus the work done by the system Copyright © The Mc. Graw-Hill Companies, Inc. Permission required for reproduction or display. 12 December 2021 University Physics, Chapter 18 20
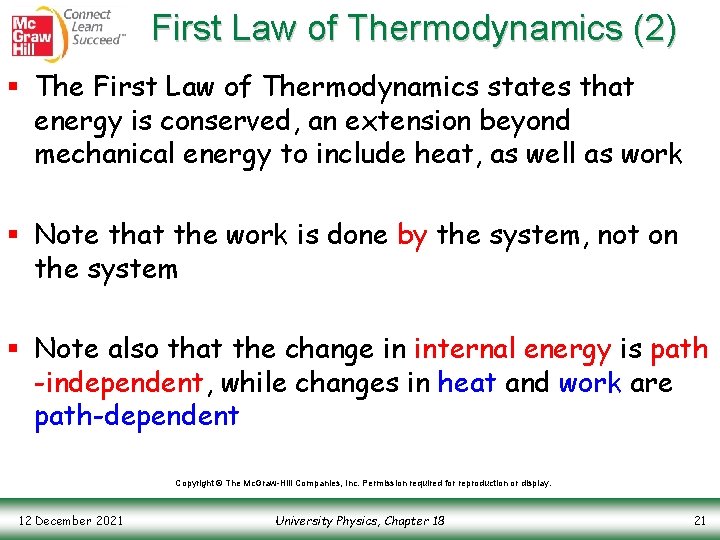
First Law of Thermodynamics (2) § The First Law of Thermodynamics states that energy is conserved, an extension beyond mechanical energy to include heat, as well as work § Note that the work is done by the system, not on the system § Note also that the change in internal energy is path -independent, while changes in heat and work are path-dependent Copyright © The Mc. Graw-Hill Companies, Inc. Permission required for reproduction or display. 12 December 2021 University Physics, Chapter 18 21
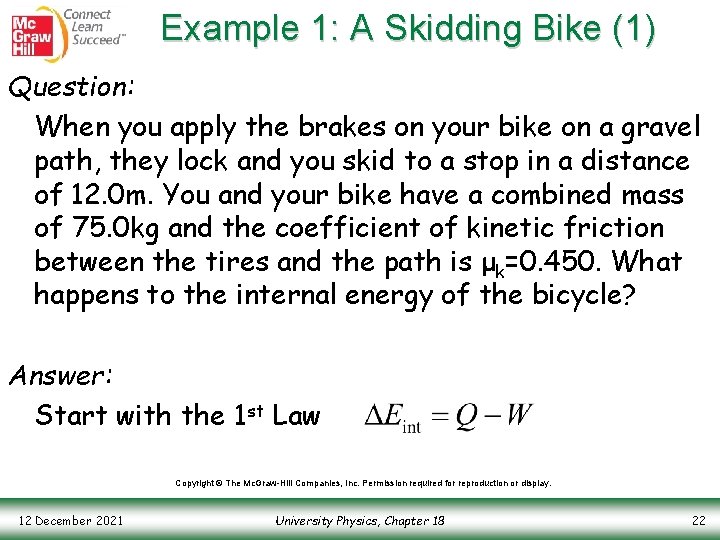
Example 1: A Skidding Bike (1) Question: When you apply the brakes on your bike on a gravel path, they lock and you skid to a stop in a distance of 12. 0 m. You and your bike have a combined mass of 75. 0 kg and the coefficient of kinetic friction between the tires and the path is μk=0. 450. What happens to the internal energy of the bicycle? Answer: Start with the 1 st Law Copyright © The Mc. Graw-Hill Companies, Inc. Permission required for reproduction or display. 12 December 2021 University Physics, Chapter 18 22
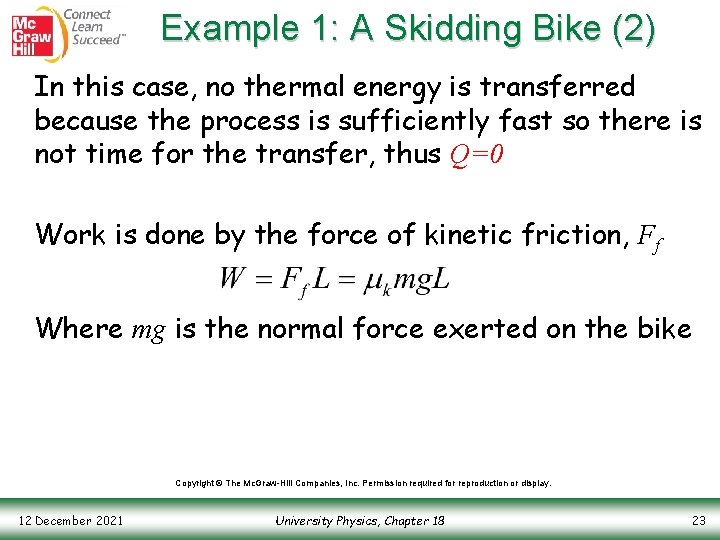
Example 1: A Skidding Bike (2) In this case, no thermal energy is transferred because the process is sufficiently fast so there is not time for the transfer, thus Q=0 Work is done by the force of kinetic friction, Ff Where mg is the normal force exerted on the bike Copyright © The Mc. Graw-Hill Companies, Inc. Permission required for reproduction or display. 12 December 2021 University Physics, Chapter 18 23
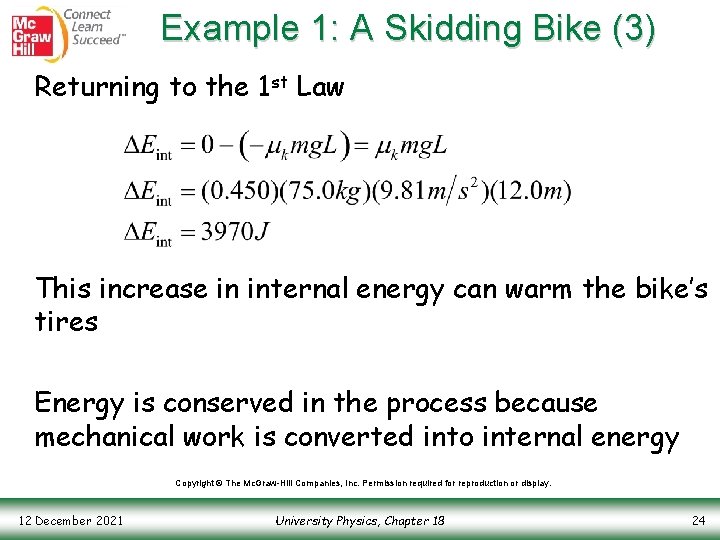
Example 1: A Skidding Bike (3) Returning to the 1 st Law This increase in internal energy can warm the bike’s tires Energy is conserved in the process because mechanical work is converted into internal energy Copyright © The Mc. Graw-Hill Companies, Inc. Permission required for reproduction or display. 12 December 2021 University Physics, Chapter 18 24
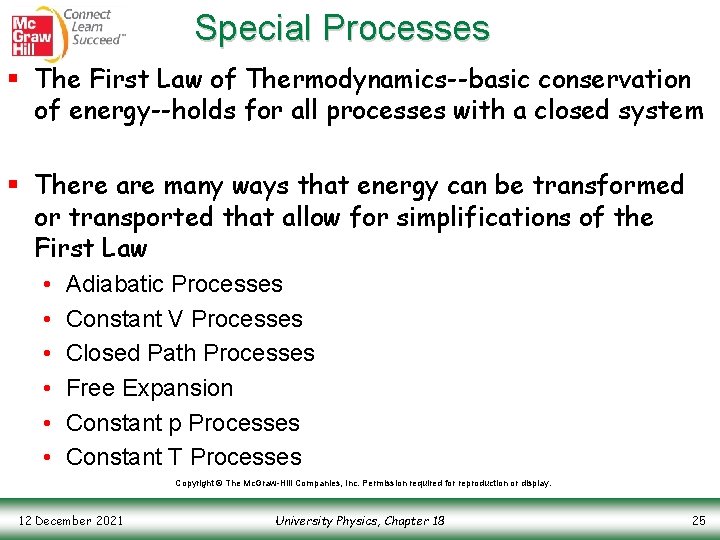
Special Processes § The First Law of Thermodynamics--basic conservation of energy--holds for all processes with a closed system § There are many ways that energy can be transformed or transported that allow for simplifications of the First Law • • • Adiabatic Processes Constant V Processes Closed Path Processes Free Expansion Constant p Processes Constant T Processes Copyright © The Mc. Graw-Hill Companies, Inc. Permission required for reproduction or display. 12 December 2021 University Physics, Chapter 18 25
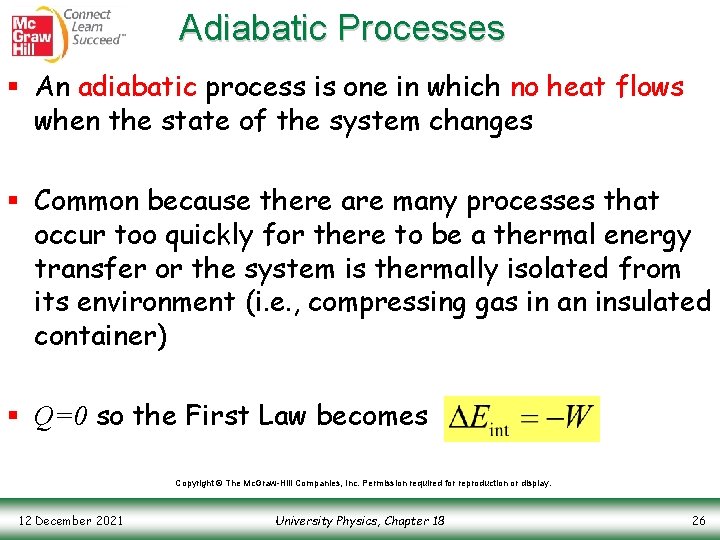
Adiabatic Processes § An adiabatic process is one in which no heat flows when the state of the system changes § Common because there are many processes that occur too quickly for there to be a thermal energy transfer or the system is thermally isolated from its environment (i. e. , compressing gas in an insulated container) § Q=0 so the First Law becomes Copyright © The Mc. Graw-Hill Companies, Inc. Permission required for reproduction or display. 12 December 2021 University Physics, Chapter 18 26
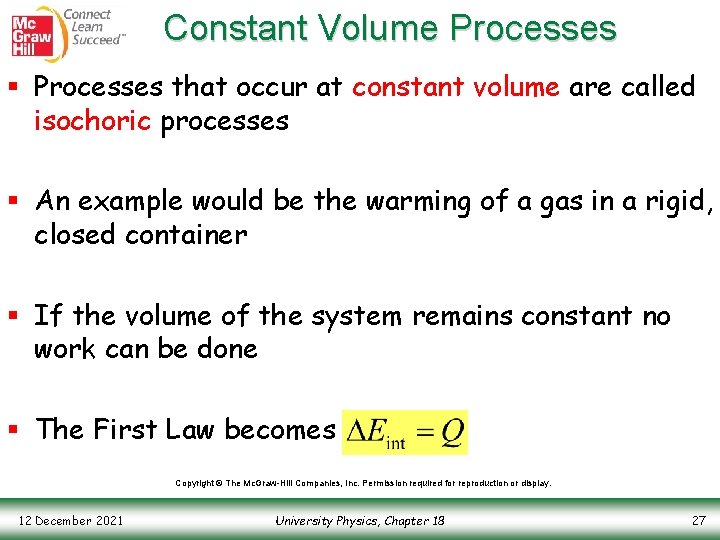
Constant Volume Processes § Processes that occur at constant volume are called isochoric processes § An example would be the warming of a gas in a rigid, closed container § If the volume of the system remains constant no work can be done § The First Law becomes Copyright © The Mc. Graw-Hill Companies, Inc. Permission required for reproduction or display. 12 December 2021 University Physics, Chapter 18 27
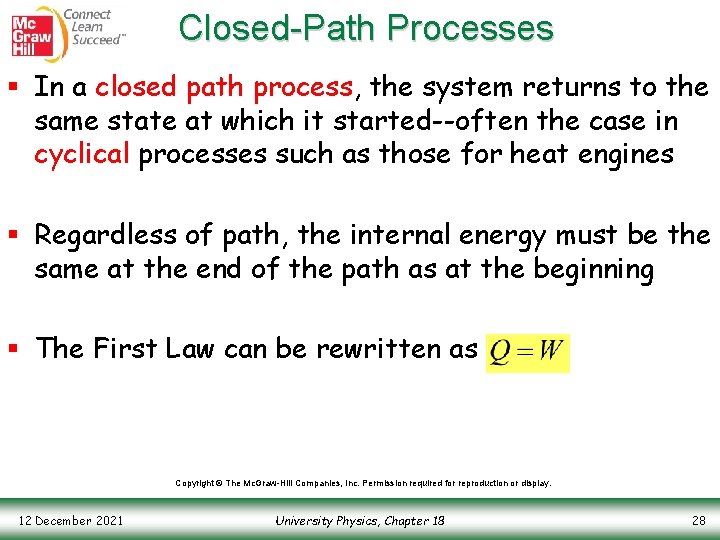
Closed-Path Processes § In a closed path process, the system returns to the same state at which it started--often the case in cyclical processes such as those for heat engines § Regardless of path, the internal energy must be the same at the end of the path as at the beginning § The First Law can be rewritten as Copyright © The Mc. Graw-Hill Companies, Inc. Permission required for reproduction or display. 12 December 2021 University Physics, Chapter 18 28
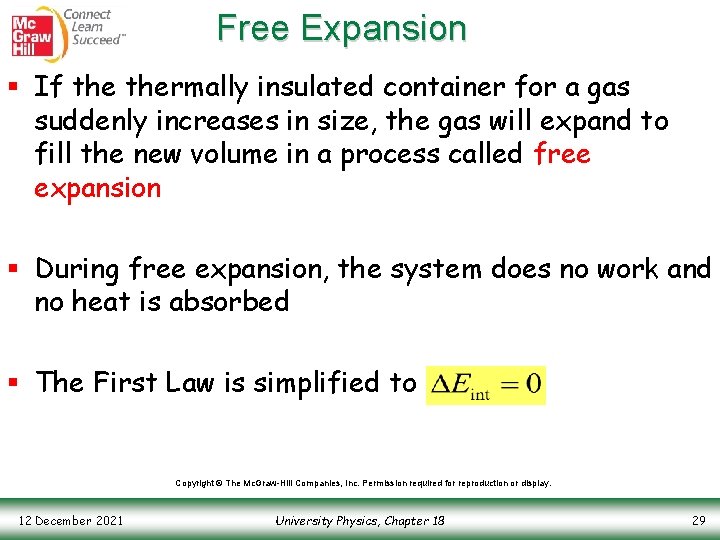
Free Expansion § If thermally insulated container for a gas suddenly increases in size, the gas will expand to fill the new volume in a process called free expansion § During free expansion, the system does no work and no heat is absorbed § The First Law is simplified to Copyright © The Mc. Graw-Hill Companies, Inc. Permission required for reproduction or display. 12 December 2021 University Physics, Chapter 18 29
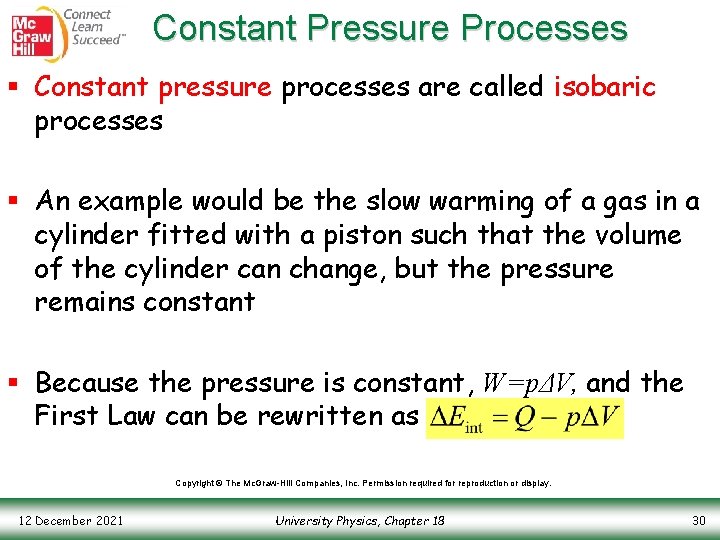
Constant Pressure Processes § Constant pressure processes are called isobaric processes § An example would be the slow warming of a gas in a cylinder fitted with a piston such that the volume of the cylinder can change, but the pressure remains constant § Because the pressure is constant, W=pΔV, and the First Law can be rewritten as Copyright © The Mc. Graw-Hill Companies, Inc. Permission required for reproduction or display. 12 December 2021 University Physics, Chapter 18 30
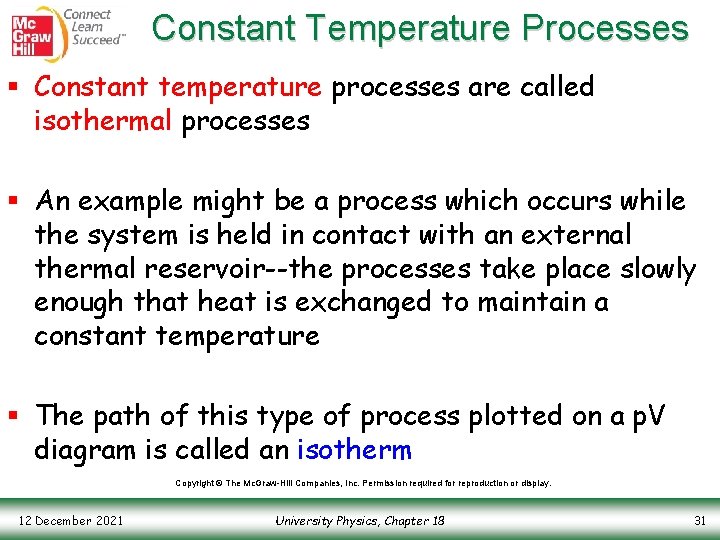
Constant Temperature Processes § Constant temperature processes are called isothermal processes § An example might be a process which occurs while the system is held in contact with an external thermal reservoir--the processes take place slowly enough that heat is exchanged to maintain a constant temperature § The path of this type of process plotted on a p. V diagram is called an isotherm Copyright © The Mc. Graw-Hill Companies, Inc. Permission required for reproduction or display. 12 December 2021 University Physics, Chapter 18 31
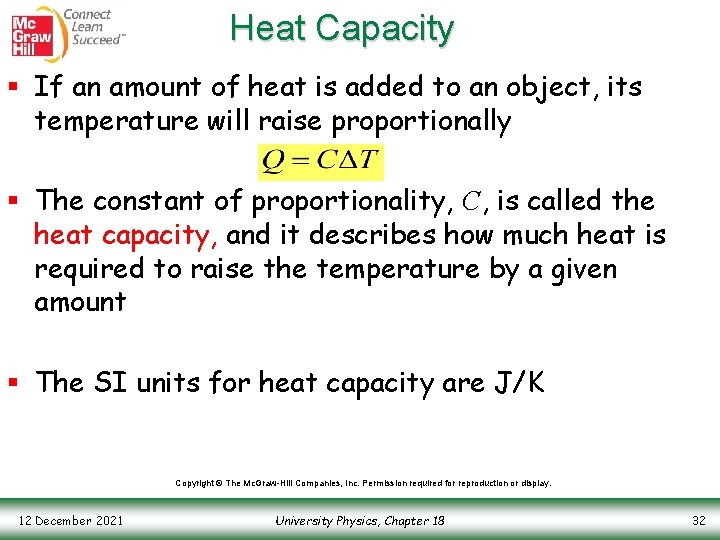
Heat Capacity § If an amount of heat is added to an object, its temperature will raise proportionally § The constant of proportionality, C, is called the heat capacity, and it describes how much heat is required to raise the temperature by a given amount § The SI units for heat capacity are J/K Copyright © The Mc. Graw-Hill Companies, Inc. Permission required for reproduction or display. 12 December 2021 University Physics, Chapter 18 32
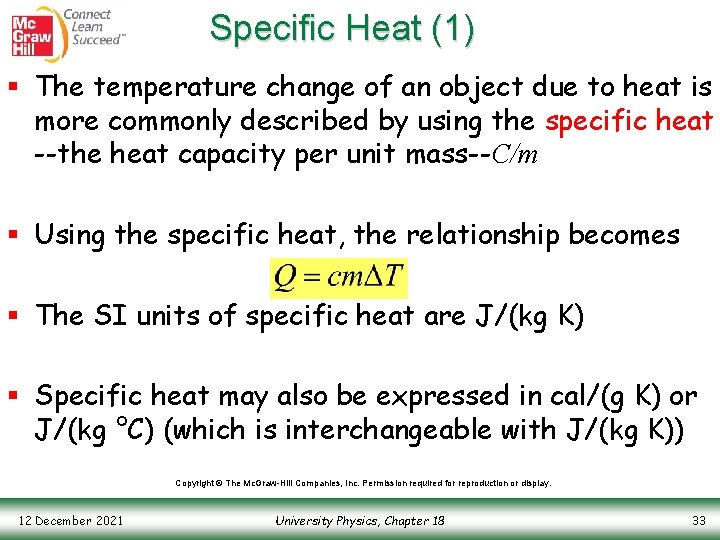
Specific Heat (1) § The temperature change of an object due to heat is more commonly described by using the specific heat --the heat capacity per unit mass--C/m § Using the specific heat, the relationship becomes § The SI units of specific heat are J/(kg K) § Specific heat may also be expressed in cal/(g K) or J/(kg °C) (which is interchangeable with J/(kg K)) Copyright © The Mc. Graw-Hill Companies, Inc. Permission required for reproduction or display. 12 December 2021 University Physics, Chapter 18 33
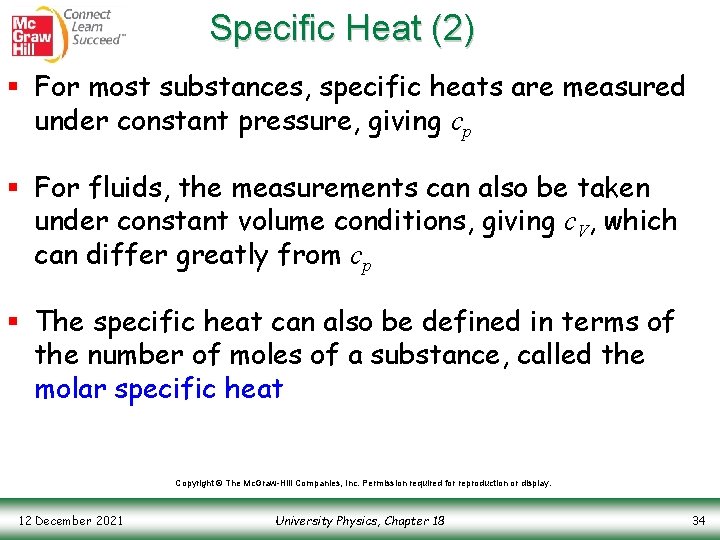
Specific Heat (2) § For most substances, specific heats are measured under constant pressure, giving cp § For fluids, the measurements can also be taken under constant volume conditions, giving c. V, which can differ greatly from cp § The specific heat can also be defined in terms of the number of moles of a substance, called the molar specific heat Copyright © The Mc. Graw-Hill Companies, Inc. Permission required for reproduction or display. 12 December 2021 University Physics, Chapter 18 34
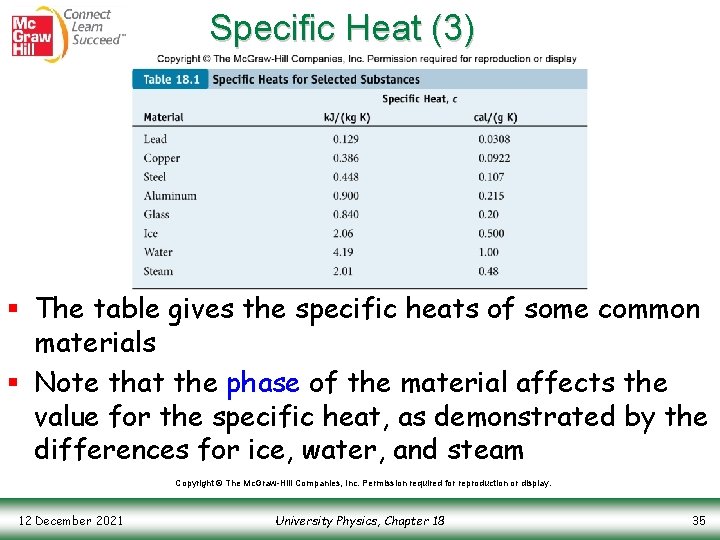
Specific Heat (3) § The table gives the specific heats of some common materials § Note that the phase of the material affects the value for the specific heat, as demonstrated by the differences for ice, water, and steam Copyright © The Mc. Graw-Hill Companies, Inc. Permission required for reproduction or display. 12 December 2021 University Physics, Chapter 18 35
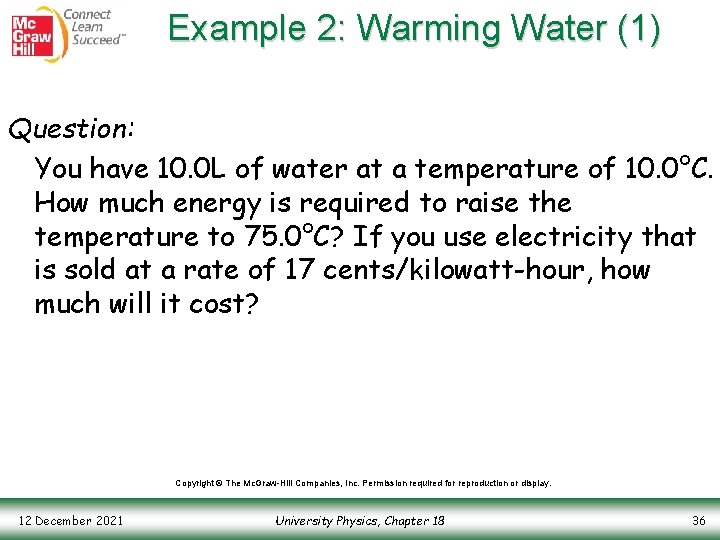
Example 2: Warming Water (1) Question: You have 10. 0 L of water at a temperature of 10. 0°C. How much energy is required to raise the temperature to 75. 0°C? If you use electricity that is sold at a rate of 17 cents/kilowatt-hour, how much will it cost? Copyright © The Mc. Graw-Hill Companies, Inc. Permission required for reproduction or display. 12 December 2021 University Physics, Chapter 18 36
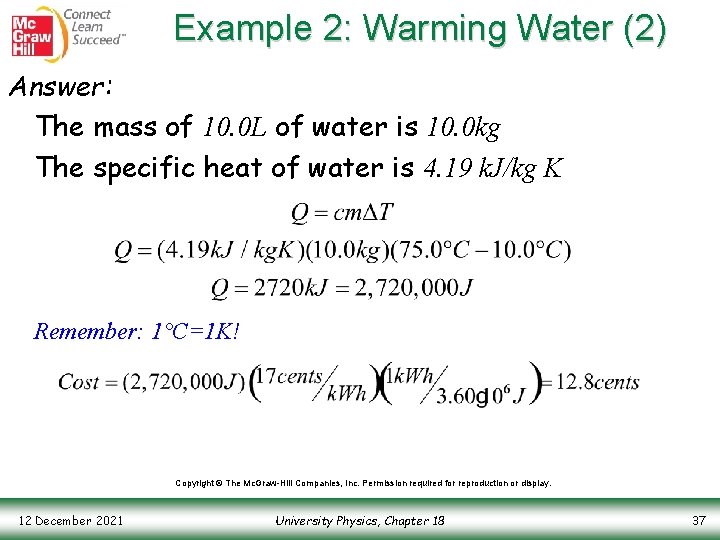
Example 2: Warming Water (2) Answer: The mass of 10. 0 L of water is 10. 0 kg The specific heat of water is 4. 19 k. J/kg K Remember: 1°C=1 K! Copyright © The Mc. Graw-Hill Companies, Inc. Permission required for reproduction or display. 12 December 2021 University Physics, Chapter 18 37
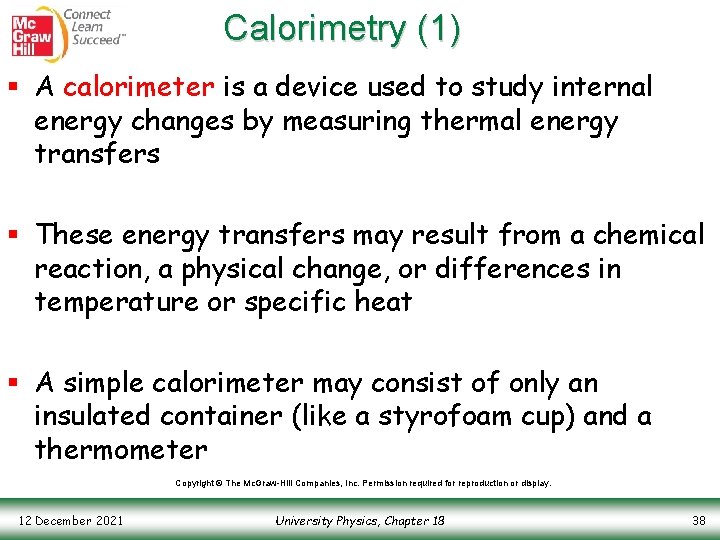
Calorimetry (1) § A calorimeter is a device used to study internal energy changes by measuring thermal energy transfers § These energy transfers may result from a chemical reaction, a physical change, or differences in temperature or specific heat § A simple calorimeter may consist of only an insulated container (like a styrofoam cup) and a thermometer Copyright © The Mc. Graw-Hill Companies, Inc. Permission required for reproduction or display. 12 December 2021 University Physics, Chapter 18 38
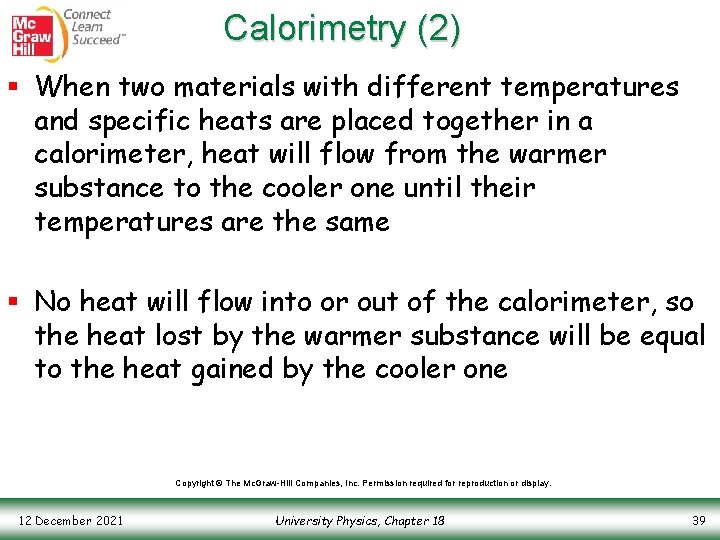
Calorimetry (2) § When two materials with different temperatures and specific heats are placed together in a calorimeter, heat will flow from the warmer substance to the cooler one until their temperatures are the same § No heat will flow into or out of the calorimeter, so the heat lost by the warmer substance will be equal to the heat gained by the cooler one Copyright © The Mc. Graw-Hill Companies, Inc. Permission required for reproduction or display. 12 December 2021 University Physics, Chapter 18 39
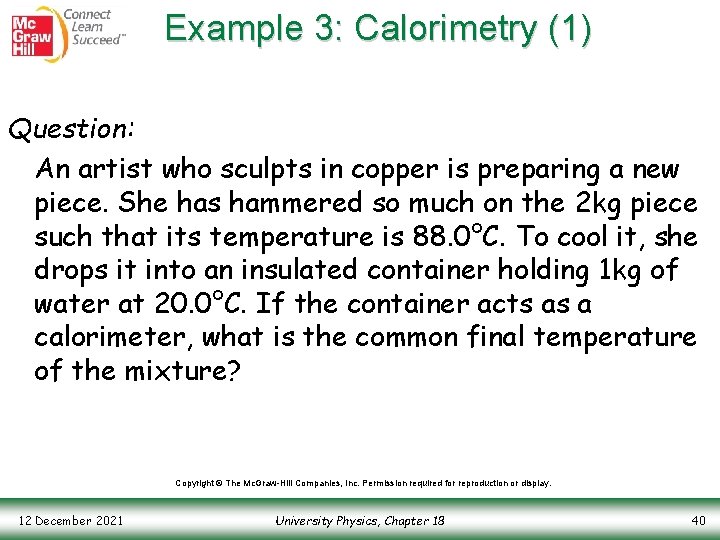
Example 3: Calorimetry (1) Question: An artist who sculpts in copper is preparing a new piece. She has hammered so much on the 2 kg piece such that its temperature is 88. 0°C. To cool it, she drops it into an insulated container holding 1 kg of water at 20. 0°C. If the container acts as a calorimeter, what is the common final temperature of the mixture? Copyright © The Mc. Graw-Hill Companies, Inc. Permission required for reproduction or display. 12 December 2021 University Physics, Chapter 18 40
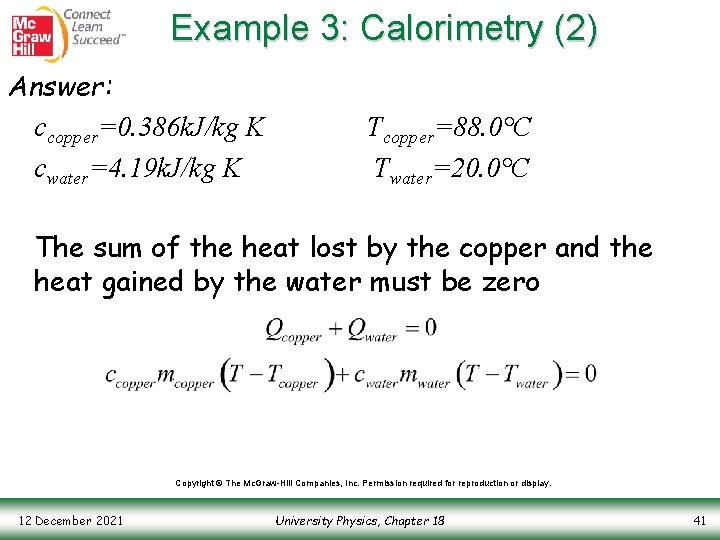
Example 3: Calorimetry (2) Answer: ccopper=0. 386 k. J/kg K cwater=4. 19 k. J/kg K Tcopper=88. 0°C Twater=20. 0°C The sum of the heat lost by the copper and the heat gained by the water must be zero Copyright © The Mc. Graw-Hill Companies, Inc. Permission required for reproduction or display. 12 December 2021 University Physics, Chapter 18 41
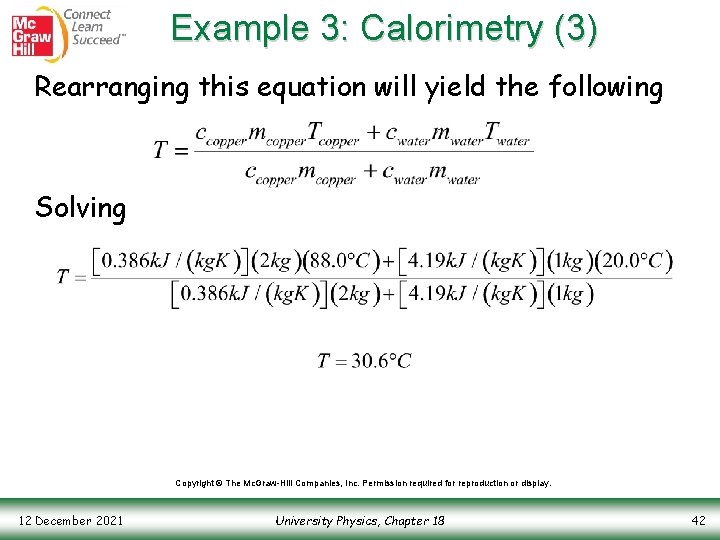
Example 3: Calorimetry (3) Rearranging this equation will yield the following Solving Copyright © The Mc. Graw-Hill Companies, Inc. Permission required for reproduction or display. 12 December 2021 University Physics, Chapter 18 42
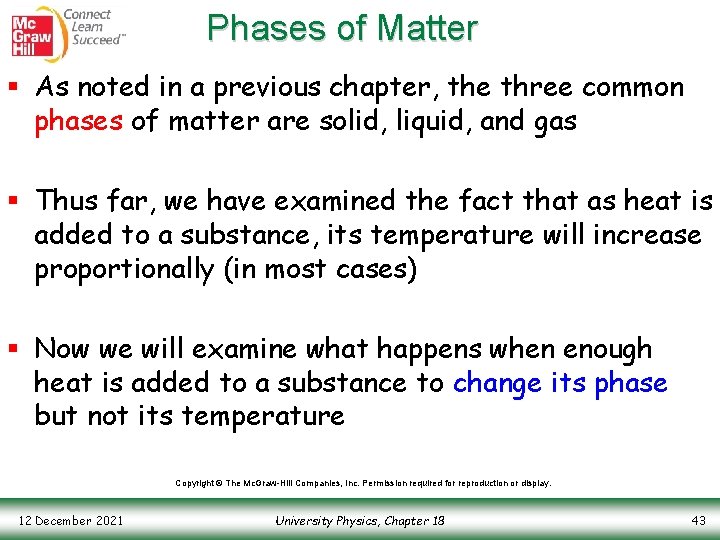
Phases of Matter § As noted in a previous chapter, the three common phases of matter are solid, liquid, and gas § Thus far, we have examined the fact that as heat is added to a substance, its temperature will increase proportionally (in most cases) § Now we will examine what happens when enough heat is added to a substance to change its phase but not its temperature Copyright © The Mc. Graw-Hill Companies, Inc. Permission required for reproduction or display. 12 December 2021 University Physics, Chapter 18 43
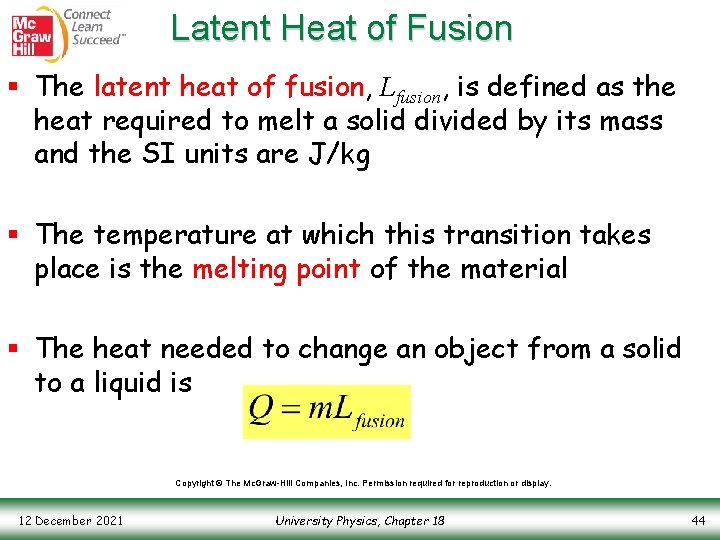
Latent Heat of Fusion § The latent heat of fusion, Lfusion, is defined as the heat required to melt a solid divided by its mass and the SI units are J/kg § The temperature at which this transition takes place is the melting point of the material § The heat needed to change an object from a solid to a liquid is Copyright © The Mc. Graw-Hill Companies, Inc. Permission required for reproduction or display. 12 December 2021 University Physics, Chapter 18 44
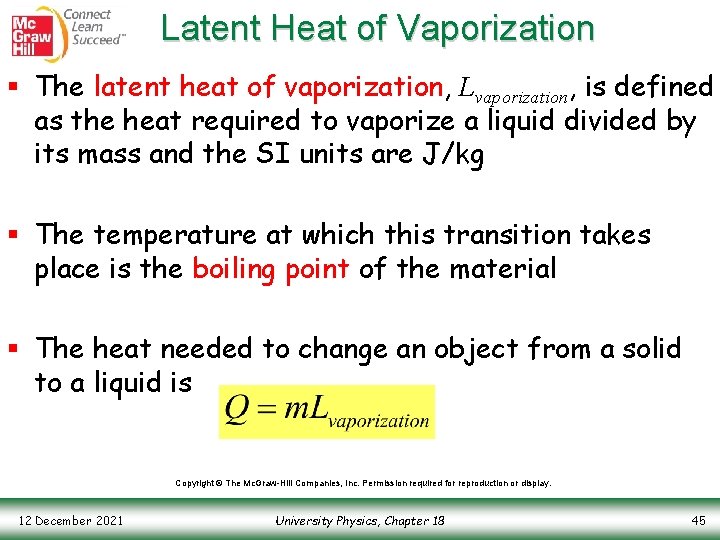
Latent Heat of Vaporization § The latent heat of vaporization, Lvaporization, is defined as the heat required to vaporize a liquid divided by its mass and the SI units are J/kg § The temperature at which this transition takes place is the boiling point of the material § The heat needed to change an object from a solid to a liquid is Copyright © The Mc. Graw-Hill Companies, Inc. Permission required for reproduction or display. 12 December 2021 University Physics, Chapter 18 45
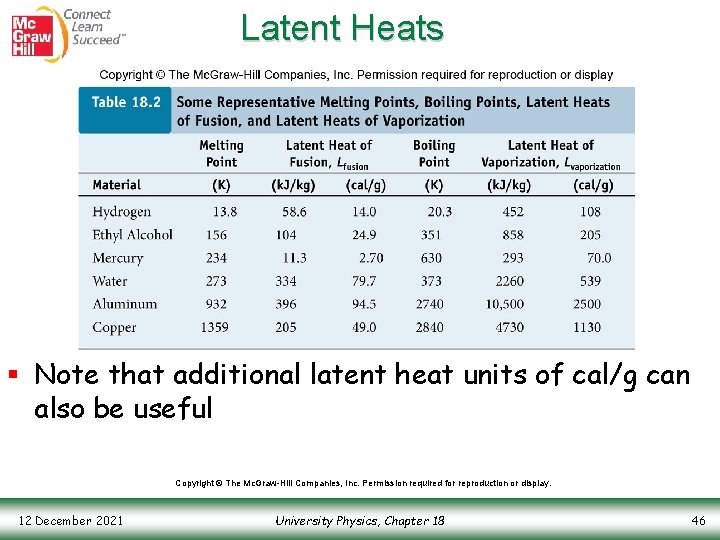
Latent Heats § Note that additional latent heat units of cal/g can also be useful Copyright © The Mc. Graw-Hill Companies, Inc. Permission required for reproduction or display. 12 December 2021 University Physics, Chapter 18 46
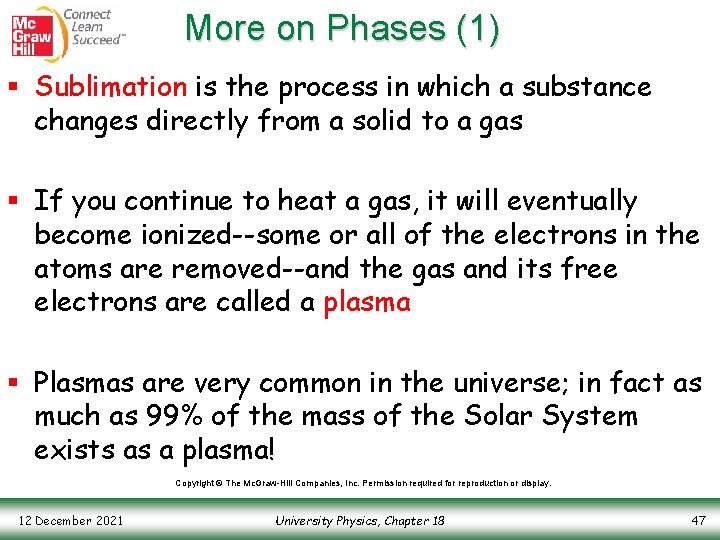
More on Phases (1) § Sublimation is the process in which a substance changes directly from a solid to a gas § If you continue to heat a gas, it will eventually become ionized--some or all of the electrons in the atoms are removed--and the gas and its free electrons are called a plasma § Plasmas are very common in the universe; in fact as much as 99% of the mass of the Solar System exists as a plasma! Copyright © The Mc. Graw-Hill Companies, Inc. Permission required for reproduction or display. 12 December 2021 University Physics, Chapter 18 47
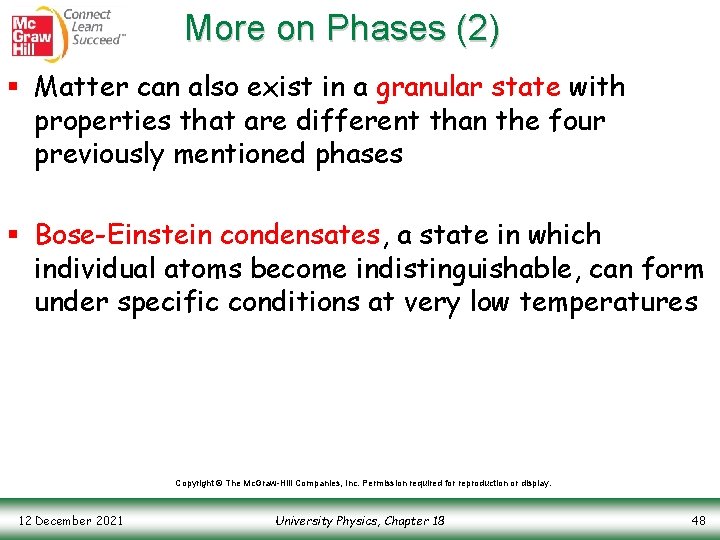
More on Phases (2) § Matter can also exist in a granular state with properties that are different than the four previously mentioned phases § Bose-Einstein condensates, a state in which individual atoms become indistinguishable, can form under specific conditions at very low temperatures Copyright © The Mc. Graw-Hill Companies, Inc. Permission required for reproduction or display. 12 December 2021 University Physics, Chapter 18 48
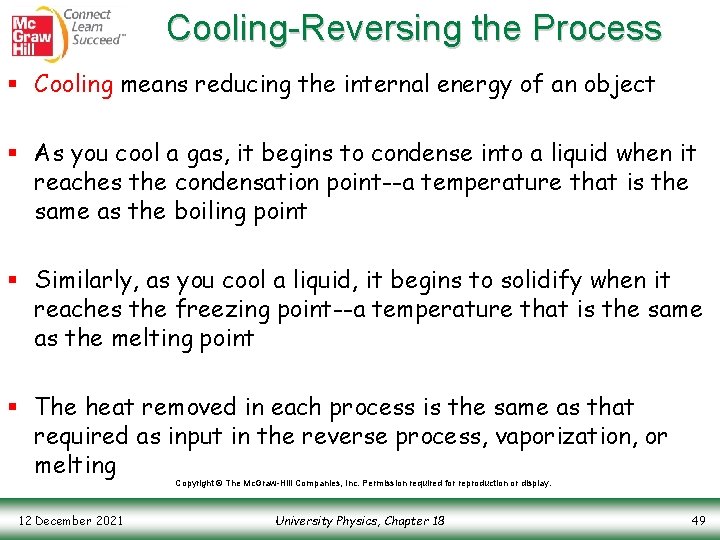
Cooling-Reversing the Process § Cooling means reducing the internal energy of an object § As you cool a gas, it begins to condense into a liquid when it reaches the condensation point--a temperature that is the same as the boiling point § Similarly, as you cool a liquid, it begins to solidify when it reaches the freezing point--a temperature that is the same as the melting point § The heat removed in each process is the same as that required as input in the reverse process, vaporization, or melting Copyright © The Mc. Graw-Hill Companies, Inc. Permission required for reproduction or display. 12 December 2021 University Physics, Chapter 18 49
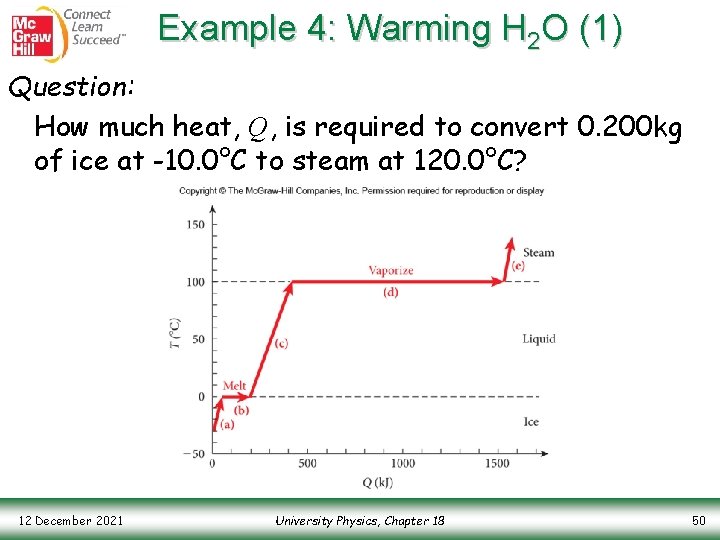
Example 4: Warming H 2 O (1) Question: How much heat, Q, is required to convert 0. 200 kg of ice at -10. 0°C to steam at 120. 0°C? Copyright © The Mc. Graw-Hill Companies, Inc. Permission required for reproduction or display. 12 December 2021 University Physics, Chapter 18 50
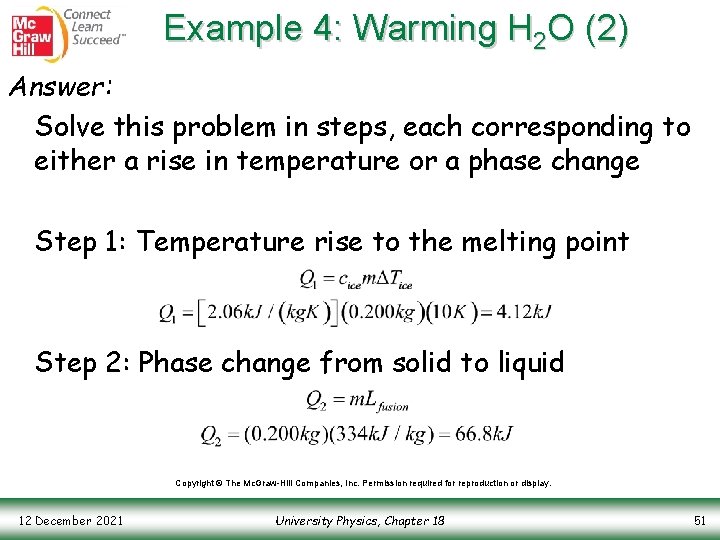
Example 4: Warming H 2 O (2) Answer: Solve this problem in steps, each corresponding to either a rise in temperature or a phase change Step 1: Temperature rise to the melting point Step 2: Phase change from solid to liquid Copyright © The Mc. Graw-Hill Companies, Inc. Permission required for reproduction or display. 12 December 2021 University Physics, Chapter 18 51
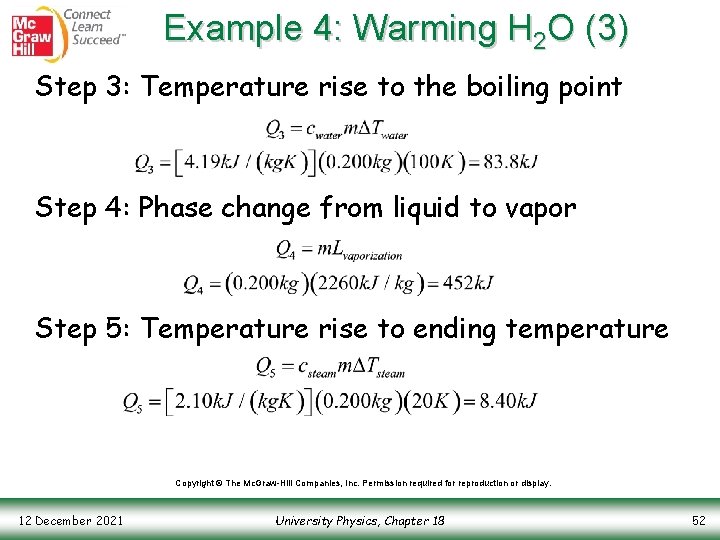
Example 4: Warming H 2 O (3) Step 3: Temperature rise to the boiling point Step 4: Phase change from liquid to vapor Step 5: Temperature rise to ending temperature Copyright © The Mc. Graw-Hill Companies, Inc. Permission required for reproduction or display. 12 December 2021 University Physics, Chapter 18 52
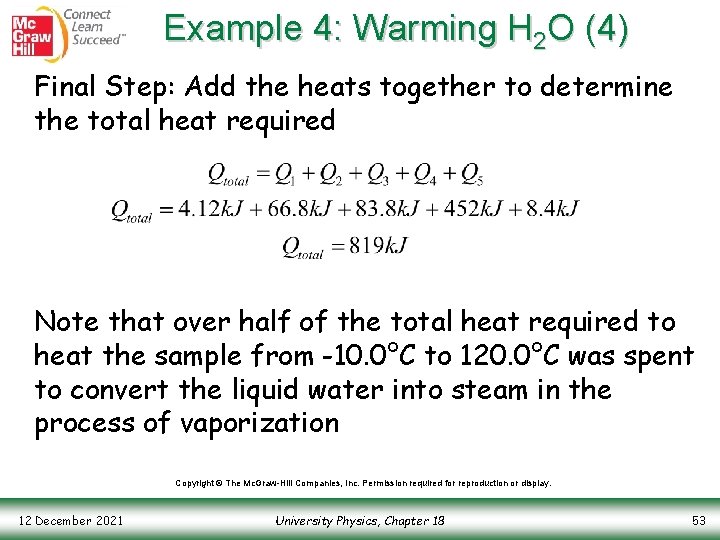
Example 4: Warming H 2 O (4) Final Step: Add the heats together to determine the total heat required Note that over half of the total heat required to heat the sample from -10. 0°C to 120. 0°C was spent to convert the liquid water into steam in the process of vaporization Copyright © The Mc. Graw-Hill Companies, Inc. Permission required for reproduction or display. 12 December 2021 University Physics, Chapter 18 53
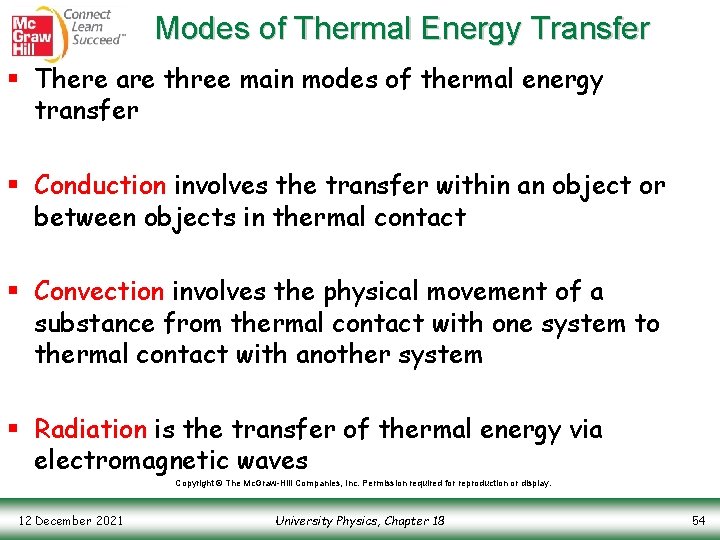
Modes of Thermal Energy Transfer § There are three main modes of thermal energy transfer § Conduction involves the transfer within an object or between objects in thermal contact § Convection involves the physical movement of a substance from thermal contact with one system to thermal contact with another system § Radiation is the transfer of thermal energy via electromagnetic waves Copyright © The Mc. Graw-Hill Companies, Inc. Permission required for reproduction or display. 12 December 2021 University Physics, Chapter 18 54
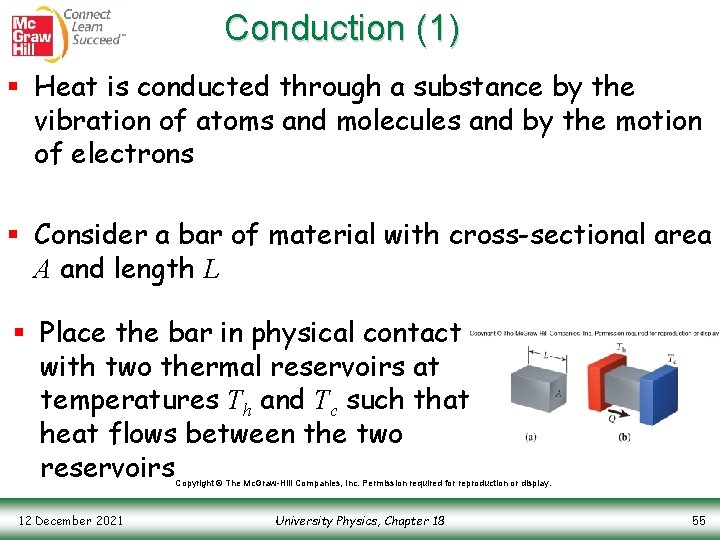
Conduction (1) § Heat is conducted through a substance by the vibration of atoms and molecules and by the motion of electrons § Consider a bar of material with cross-sectional area A and length L § Place the bar in physical contact with two thermal reservoirs at temperatures Th and Tc such that heat flows between the two reservoirs Copyright © The Mc. Graw-Hill Companies, Inc. Permission required for reproduction or display. 12 December 2021 University Physics, Chapter 18 55
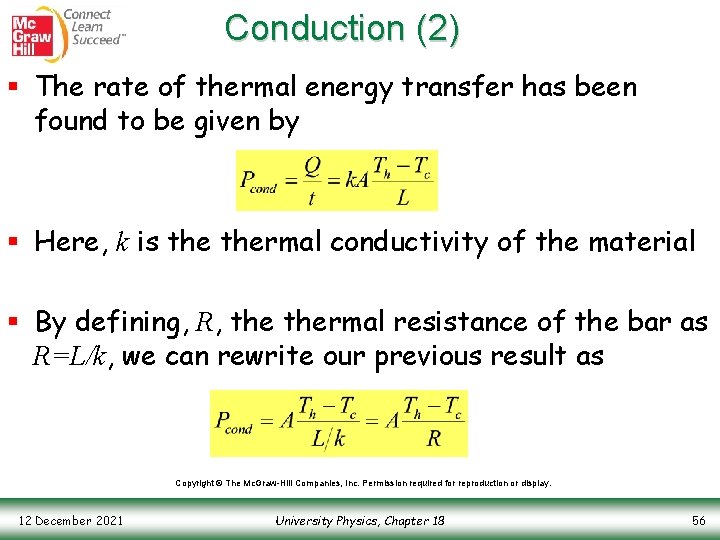
Conduction (2) § The rate of thermal energy transfer has been found to be given by § Here, k is thermal conductivity of the material § By defining, R, thermal resistance of the bar as R=L/k, we can rewrite our previous result as Copyright © The Mc. Graw-Hill Companies, Inc. Permission required for reproduction or display. 12 December 2021 University Physics, Chapter 18 56
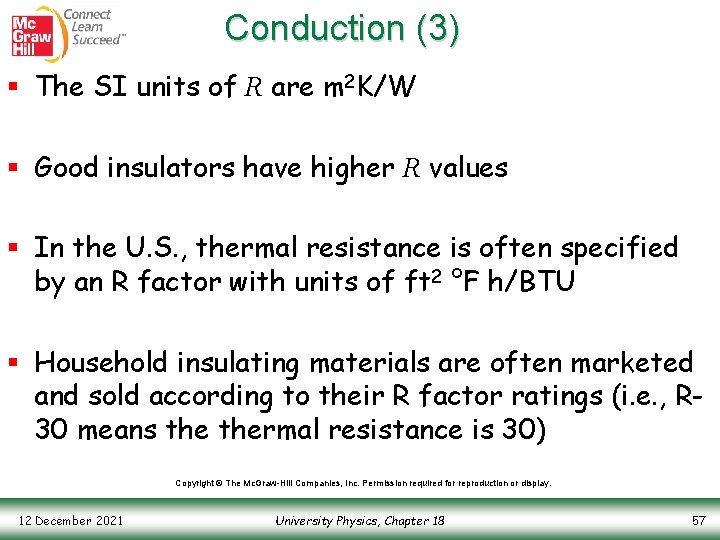
Conduction (3) § The SI units of R are m 2 K/W § Good insulators have higher R values § In the U. S. , thermal resistance is often specified by an R factor with units of ft 2 °F h/BTU § Household insulating materials are often marketed and sold according to their R factor ratings (i. e. , R 30 means thermal resistance is 30) Copyright © The Mc. Graw-Hill Companies, Inc. Permission required for reproduction or display. 12 December 2021 University Physics, Chapter 18 57
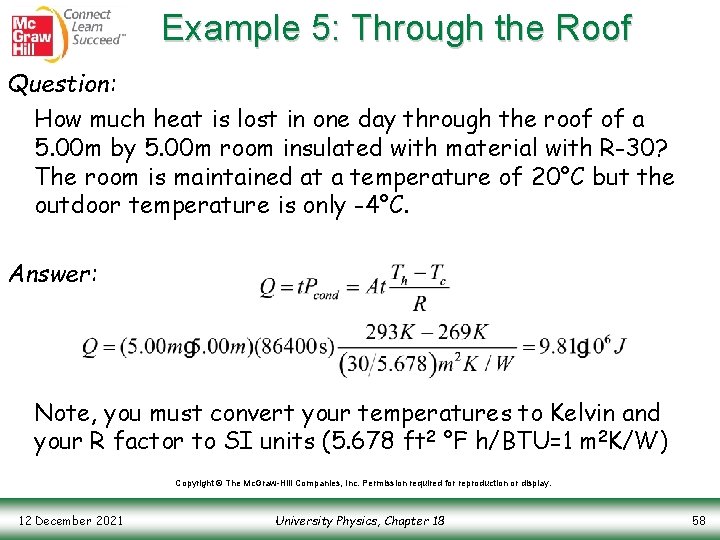
Example 5: Through the Roof Question: How much heat is lost in one day through the roof of a 5. 00 m by 5. 00 m room insulated with material with R-30? The room is maintained at a temperature of 20°C but the outdoor temperature is only -4°C. Answer: Note, you must convert your temperatures to Kelvin and your R factor to SI units (5. 678 ft 2 °F h/BTU=1 m 2 K/W) Copyright © The Mc. Graw-Hill Companies, Inc. Permission required for reproduction or display. 12 December 2021 University Physics, Chapter 18 58
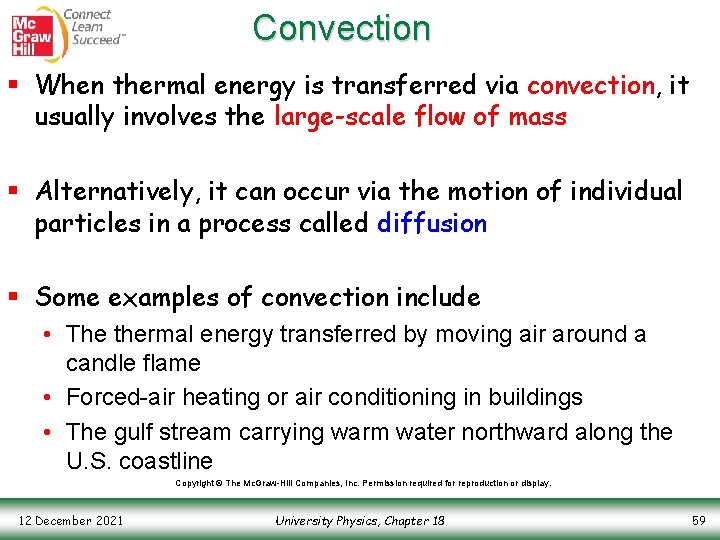
Convection § When thermal energy is transferred via convection, it usually involves the large-scale flow of mass § Alternatively, it can occur via the motion of individual particles in a process called diffusion § Some examples of convection include • The thermal energy transferred by moving air around a candle flame • Forced-air heating or air conditioning in buildings • The gulf stream carrying warm water northward along the U. S. coastline Copyright © The Mc. Graw-Hill Companies, Inc. Permission required for reproduction or display. 12 December 2021 University Physics, Chapter 18 59
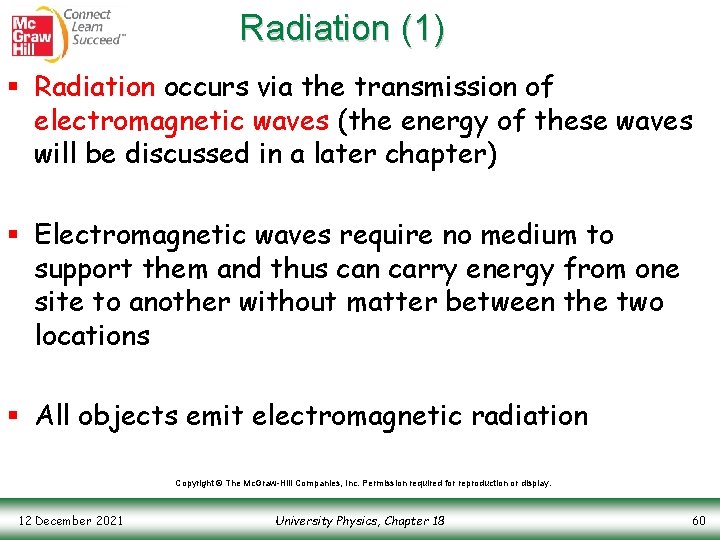
Radiation (1) § Radiation occurs via the transmission of electromagnetic waves (the energy of these waves will be discussed in a later chapter) § Electromagnetic waves require no medium to support them and thus can carry energy from one site to another without matter between the two locations § All objects emit electromagnetic radiation Copyright © The Mc. Graw-Hill Companies, Inc. Permission required for reproduction or display. 12 December 2021 University Physics, Chapter 18 60
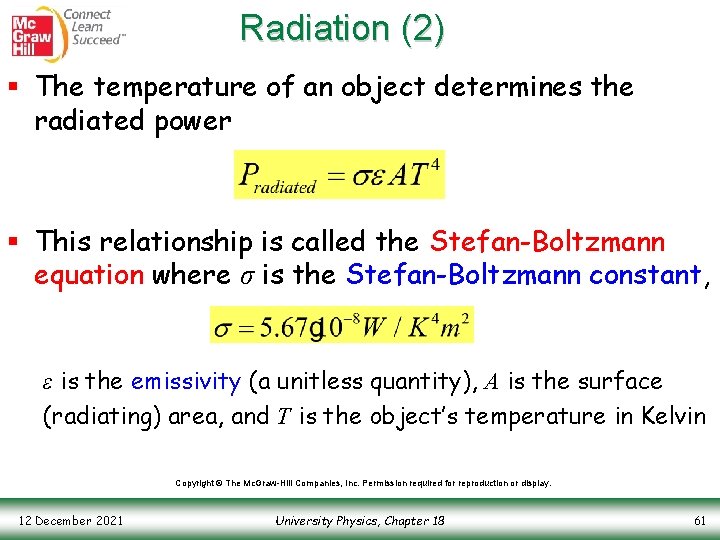
Radiation (2) § The temperature of an object determines the radiated power § This relationship is called the Stefan-Boltzmann equation where σ is the Stefan-Boltzmann constant, ε is the emissivity (a unitless quantity), A is the surface (radiating) area, and T is the object’s temperature in Kelvin Copyright © The Mc. Graw-Hill Companies, Inc. Permission required for reproduction or display. 12 December 2021 University Physics, Chapter 18 61
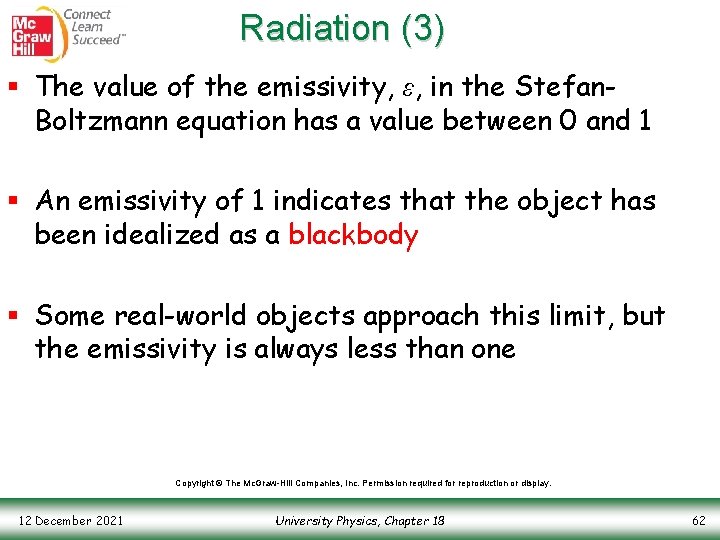
Radiation (3) § The value of the emissivity, ε, in the Stefan. Boltzmann equation has a value between 0 and 1 § An emissivity of 1 indicates that the object has been idealized as a blackbody § Some real-world objects approach this limit, but the emissivity is always less than one Copyright © The Mc. Graw-Hill Companies, Inc. Permission required for reproduction or display. 12 December 2021 University Physics, Chapter 18 62
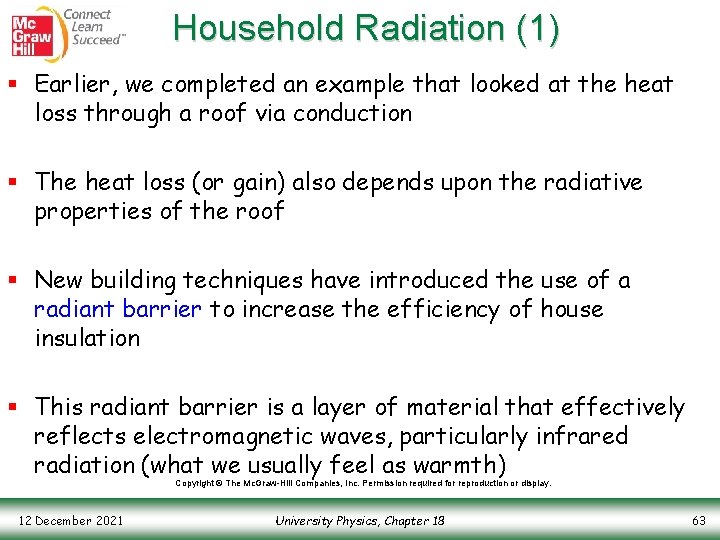
Household Radiation (1) § Earlier, we completed an example that looked at the heat loss through a roof via conduction § The heat loss (or gain) also depends upon the radiative properties of the roof § New building techniques have introduced the use of a radiant barrier to increase the efficiency of house insulation § This radiant barrier is a layer of material that effectively reflects electromagnetic waves, particularly infrared radiation (what we usually feel as warmth) Copyright © The Mc. Graw-Hill Companies, Inc. Permission required for reproduction or display. 12 December 2021 University Physics, Chapter 18 63
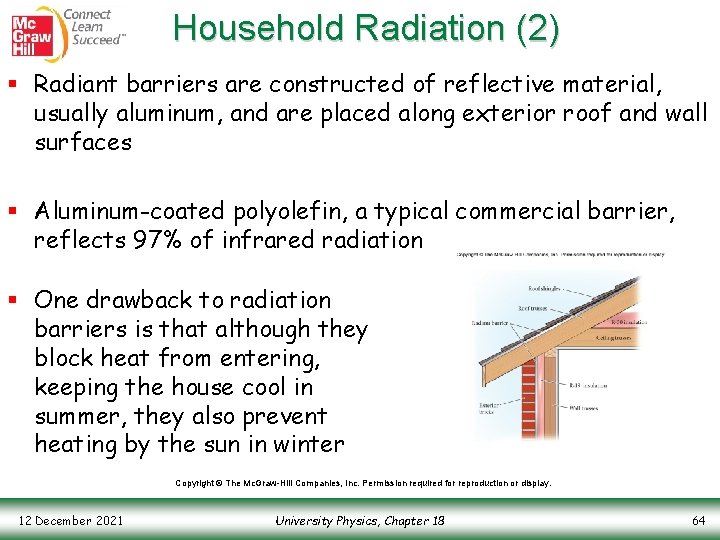
Household Radiation (2) § Radiant barriers are constructed of reflective material, usually aluminum, and are placed along exterior roof and wall surfaces § Aluminum-coated polyolefin, a typical commercial barrier, reflects 97% of infrared radiation § One drawback to radiation barriers is that although they block heat from entering, keeping the house cool in summer, they also prevent heating by the sun in winter Copyright © The Mc. Graw-Hill Companies, Inc. Permission required for reproduction or display. 12 December 2021 University Physics, Chapter 18 64
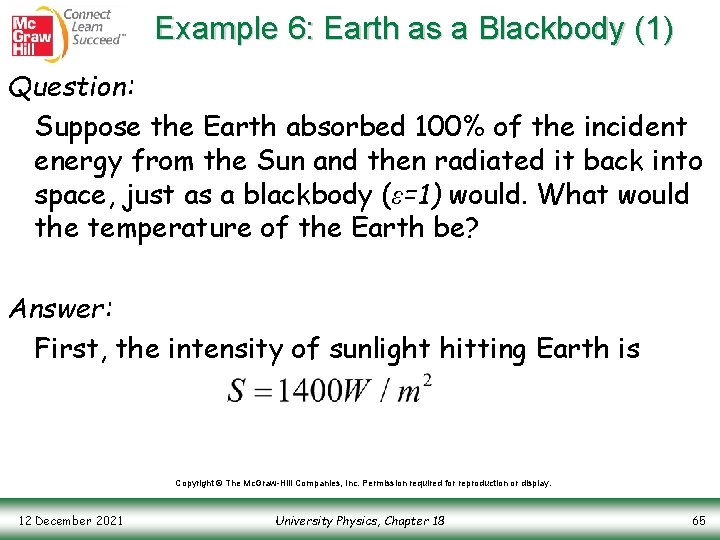
Example 6: Earth as a Blackbody (1) Question: Suppose the Earth absorbed 100% of the incident energy from the Sun and then radiated it back into space, just as a blackbody (ε=1) would. What would the temperature of the Earth be? Answer: First, the intensity of sunlight hitting Earth is Copyright © The Mc. Graw-Hill Companies, Inc. Permission required for reproduction or display. 12 December 2021 University Physics, Chapter 18 65
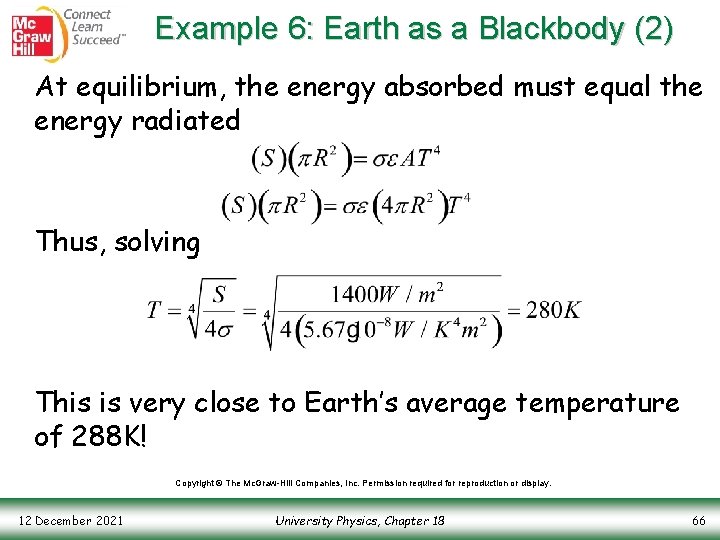
Example 6: Earth as a Blackbody (2) At equilibrium, the energy absorbed must equal the energy radiated Thus, solving This is very close to Earth’s average temperature of 288 K! Copyright © The Mc. Graw-Hill Companies, Inc. Permission required for reproduction or display. 12 December 2021 University Physics, Chapter 18 66
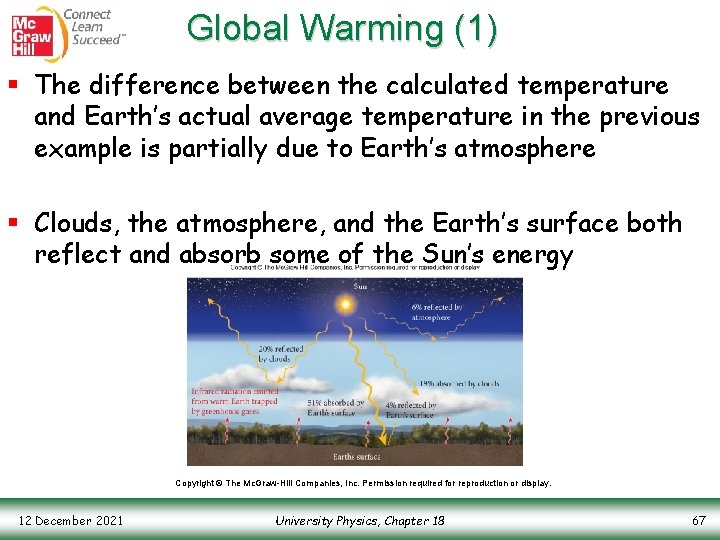
Global Warming (1) § The difference between the calculated temperature and Earth’s actual average temperature in the previous example is partially due to Earth’s atmosphere § Clouds, the atmosphere, and the Earth’s surface both reflect and absorb some of the Sun’s energy Copyright © The Mc. Graw-Hill Companies, Inc. Permission required for reproduction or display. 12 December 2021 University Physics, Chapter 18 67
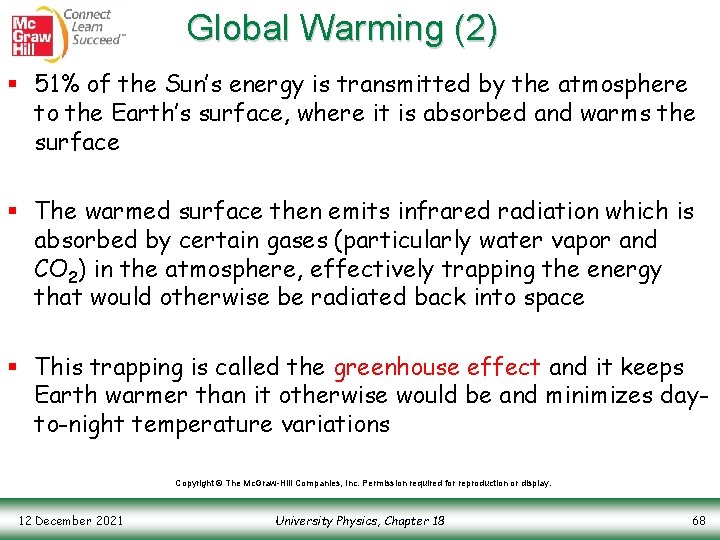
Global Warming (2) § 51% of the Sun’s energy is transmitted by the atmosphere to the Earth’s surface, where it is absorbed and warms the surface § The warmed surface then emits infrared radiation which is absorbed by certain gases (particularly water vapor and CO 2) in the atmosphere, effectively trapping the energy that would otherwise be radiated back into space § This trapping is called the greenhouse effect and it keeps Earth warmer than it otherwise would be and minimizes dayto-night temperature variations Copyright © The Mc. Graw-Hill Companies, Inc. Permission required for reproduction or display. 12 December 2021 University Physics, Chapter 18 68
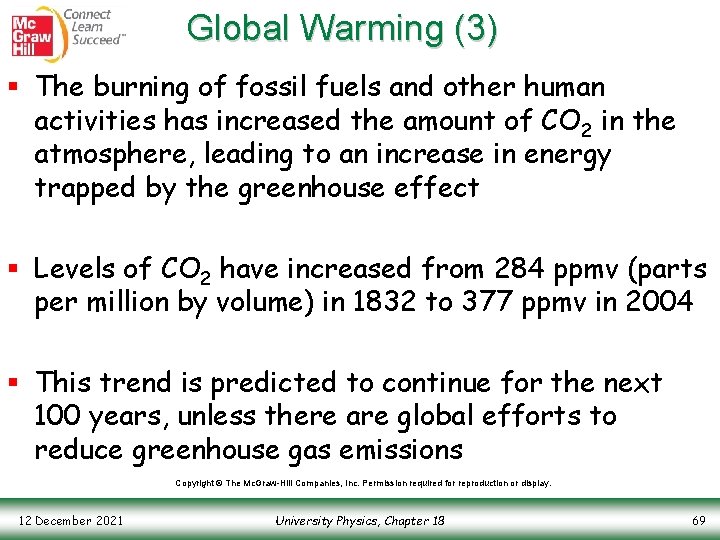
Global Warming (3) § The burning of fossil fuels and other human activities has increased the amount of CO 2 in the atmosphere, leading to an increase in energy trapped by the greenhouse effect § Levels of CO 2 have increased from 284 ppmv (parts per million by volume) in 1832 to 377 ppmv in 2004 § This trend is predicted to continue for the next 100 years, unless there are global efforts to reduce greenhouse gas emissions Copyright © The Mc. Graw-Hill Companies, Inc. Permission required for reproduction or display. 12 December 2021 University Physics, Chapter 18 69
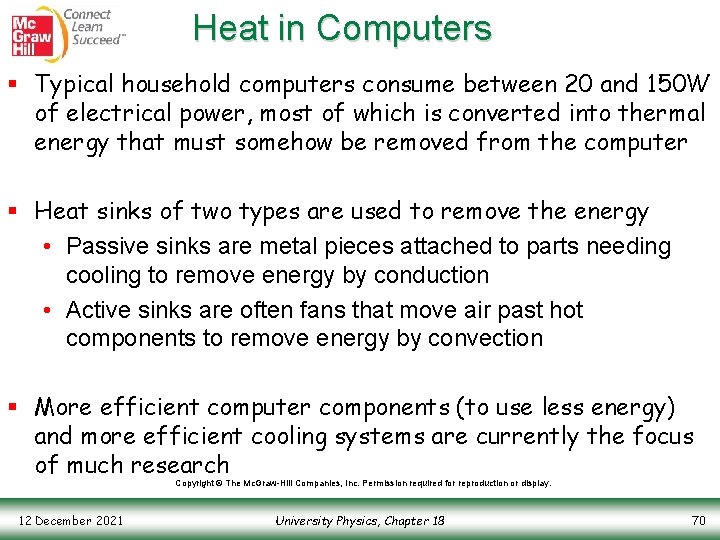
Heat in Computers § Typical household computers consume between 20 and 150 W of electrical power, most of which is converted into thermal energy that must somehow be removed from the computer § Heat sinks of two types are used to remove the energy • Passive sinks are metal pieces attached to parts needing cooling to remove energy by conduction • Active sinks are often fans that move air past hot components to remove energy by convection § More efficient computer components (to use less energy) and more efficient cooling systems are currently the focus of much research Copyright © The Mc. Graw-Hill Companies, Inc. Permission required for reproduction or display. 12 December 2021 University Physics, Chapter 18 70
How is heat energy transferred
Whats conduction convection and radiation
3 ways heat is transferred
Heat energy
How is heat transferred in the mantle
Conduction or convection
How is energy transferred within the water cycle
Energy transferred equation
Trophic level transfer efficiency formula
During conduction, thermal energy is transferred
Thermal energy vs heat energy
Energy energy transfer and general energy analysis
Energy energy transfer and general energy analysis
Techniques of transferring pattern markings on the fabric
Transferred epithet figure of speech
A spoon in a cup of hot soup becomes warmer
Transferred epithet figure of speech
In a redox reaction, electrons are transferred
Self pollination
Personification in on the grasshopper and the cricket
Transferred epithet
Male and female part of a flower
Standard hydrogen electrode conditions
Transfer of malice
How to calculate cost of goods transferred out
Idiomatic diction
Transferred epithet
Transferred epithet
Plasma transferred arc welding equipment
How to calculate cost of goods transferred out
Transfer of command briefing
Jami lost her debit card
Which is a tcs food bread flour sprouts strawberries
Hình ảnh bộ gõ cơ thể búng tay
Slidetodoc
Bổ thể
Tỉ lệ cơ thể trẻ em
Gấu đi như thế nào
Chụp tư thế worms-breton
Chúa yêu trần thế
Các môn thể thao bắt đầu bằng từ đua
Thế nào là hệ số cao nhất
Các châu lục và đại dương trên thế giới
Công thức tiính động năng
Trời xanh đây là của chúng ta thể thơ
Mật thư tọa độ 5x5
101012 bằng
độ dài liên kết
Các châu lục và đại dương trên thế giới
Thơ thất ngôn tứ tuyệt đường luật
Quá trình desamine hóa có thể tạo ra
Một số thể thơ truyền thống
Cái miệng nó xinh thế chỉ nói điều hay thôi
Vẽ hình chiếu vuông góc của vật thể sau
Nguyên nhân của sự mỏi cơ sinh 8
đặc điểm cơ thể của người tối cổ
V. c c
Vẽ hình chiếu đứng bằng cạnh của vật thể
Phối cảnh
Thẻ vin
đại từ thay thế
điện thế nghỉ
Tư thế ngồi viết
Diễn thế sinh thái là
Các loại đột biến cấu trúc nhiễm sắc thể
Thế nào là số nguyên tố
Tư thế ngồi viết
Lời thề hippocrates
Thiếu nhi thế giới liên hoan
ưu thế lai là gì
Khi nào hổ con có thể sống độc lập