The Formation of Massive Stars and Star Clusters
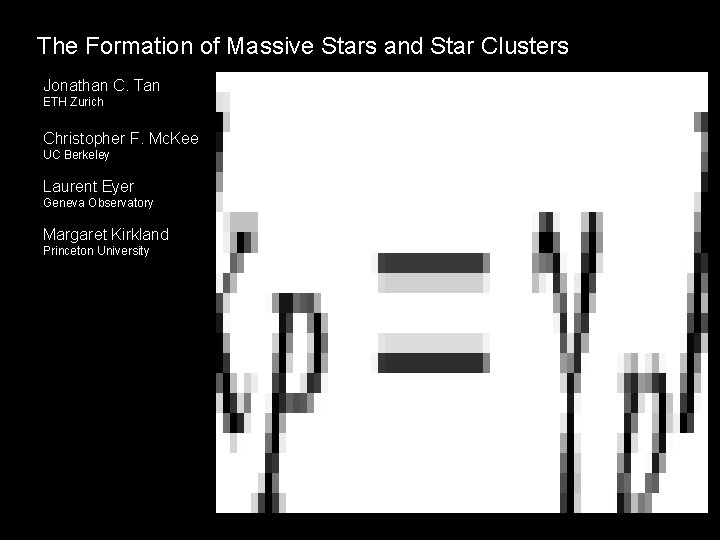
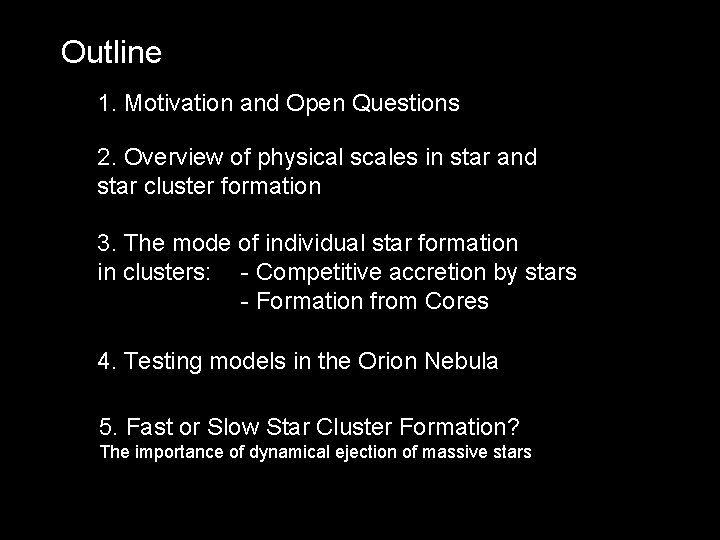
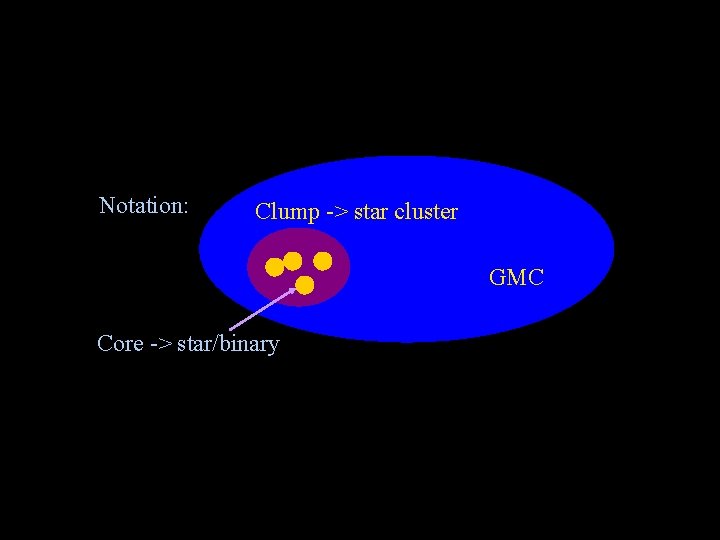
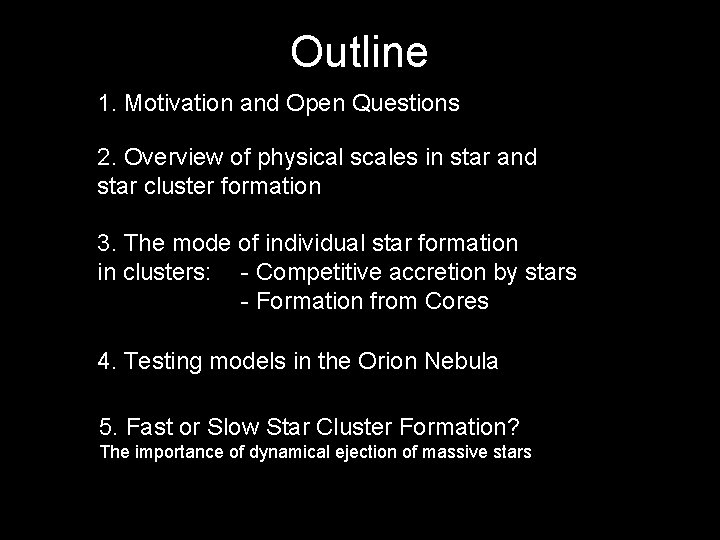
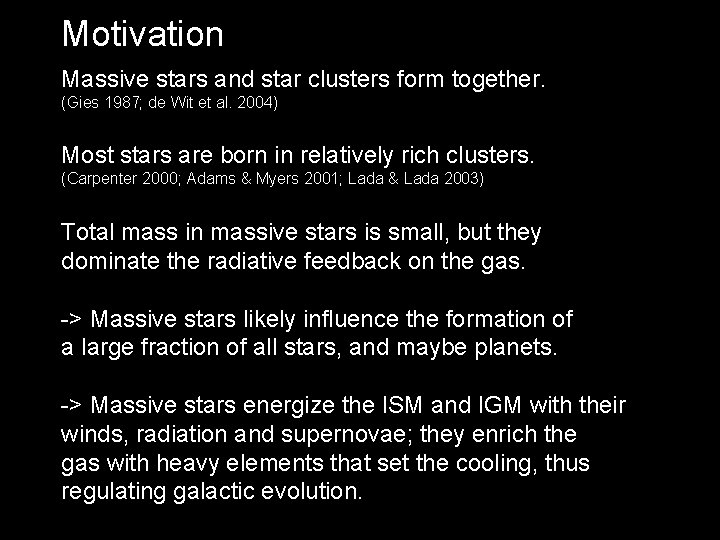
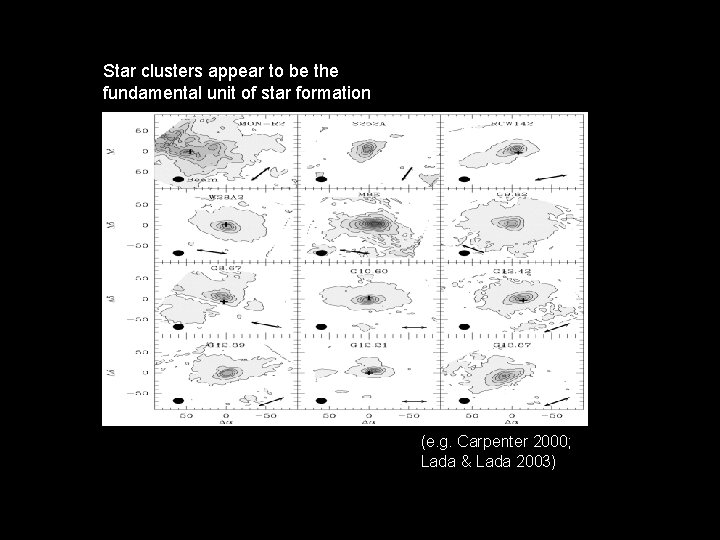
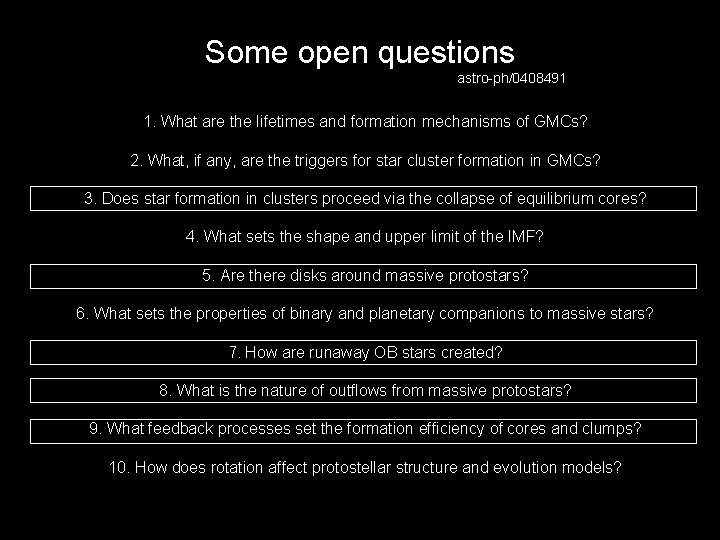
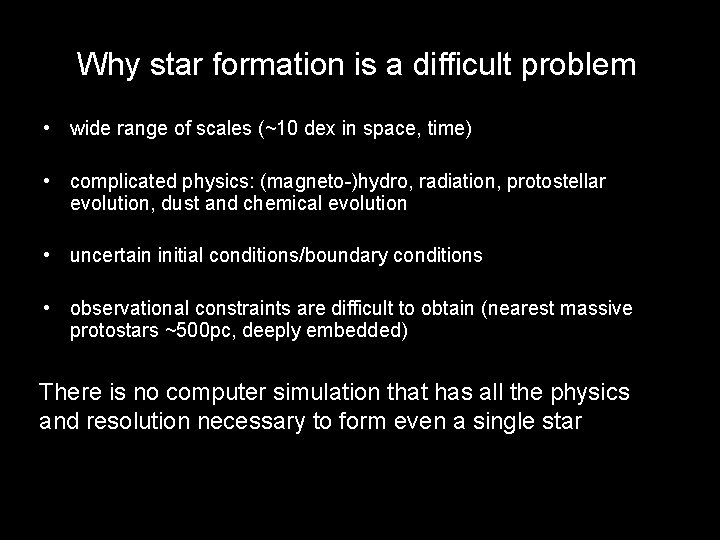
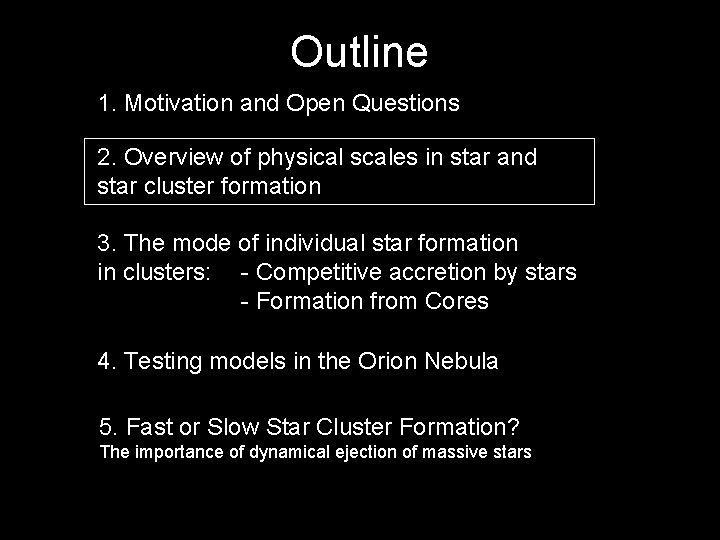
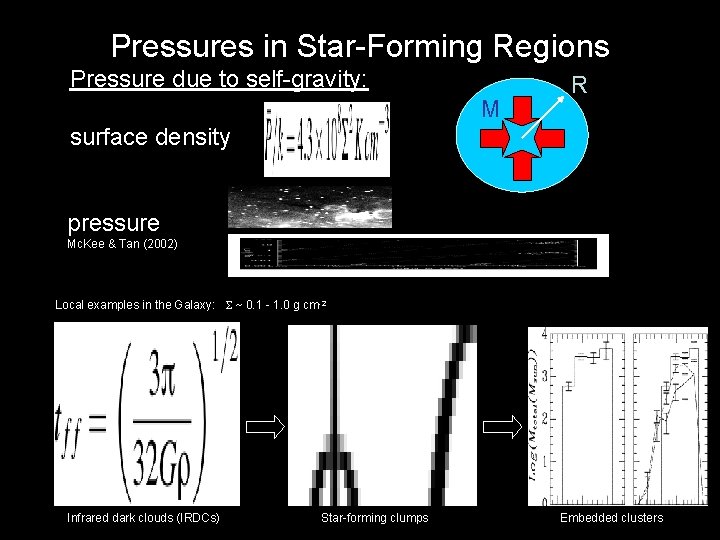
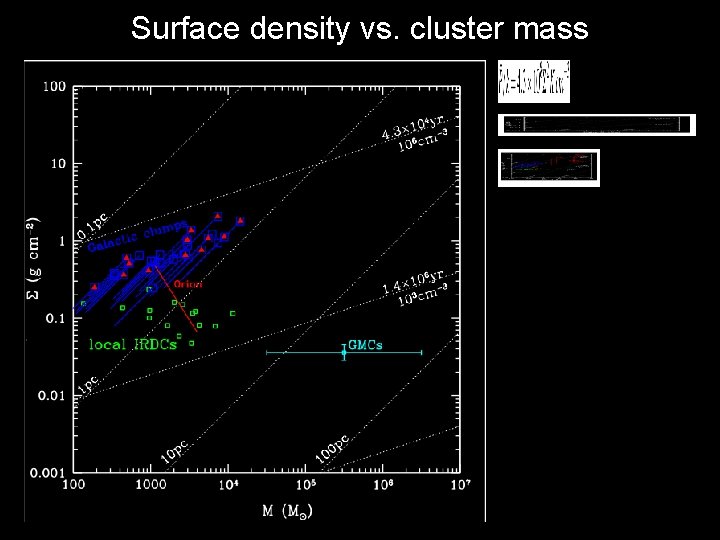
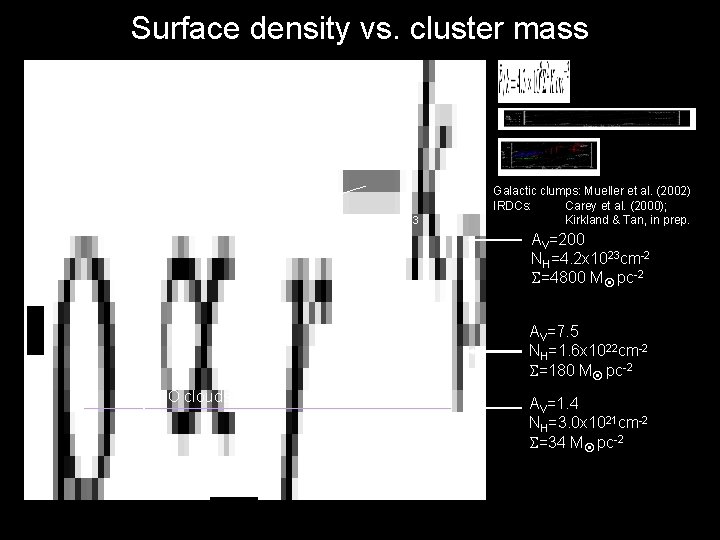
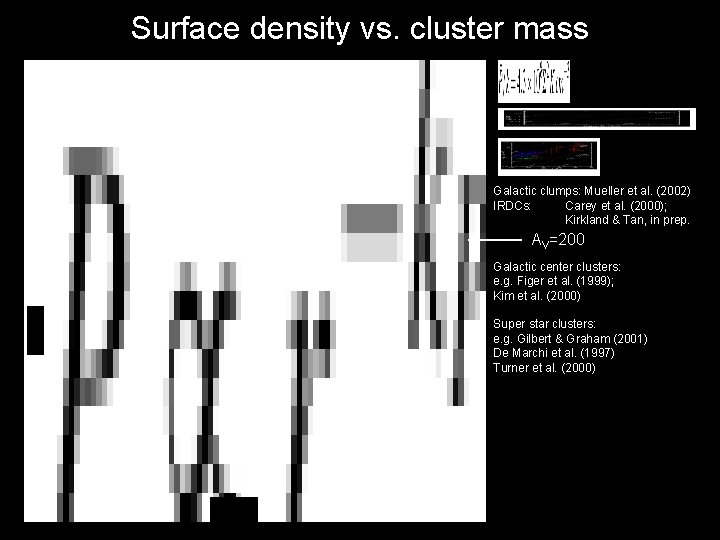
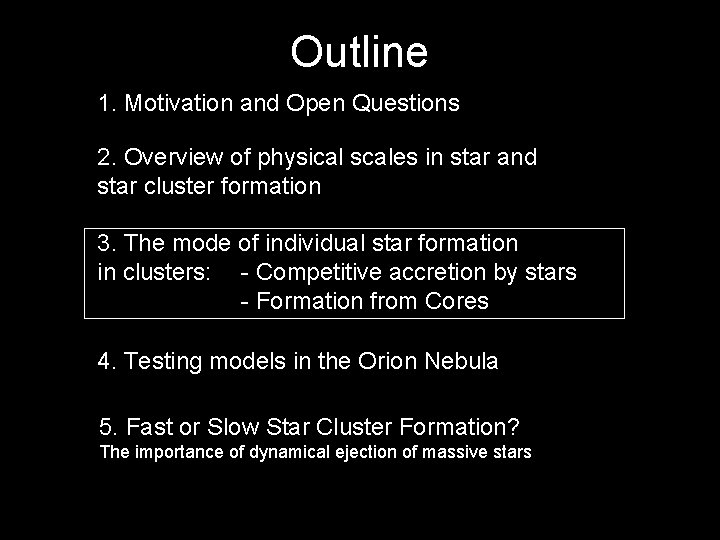
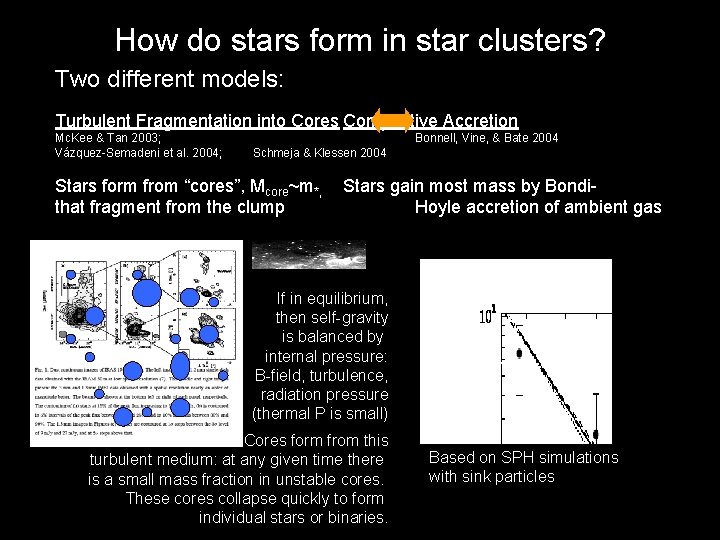
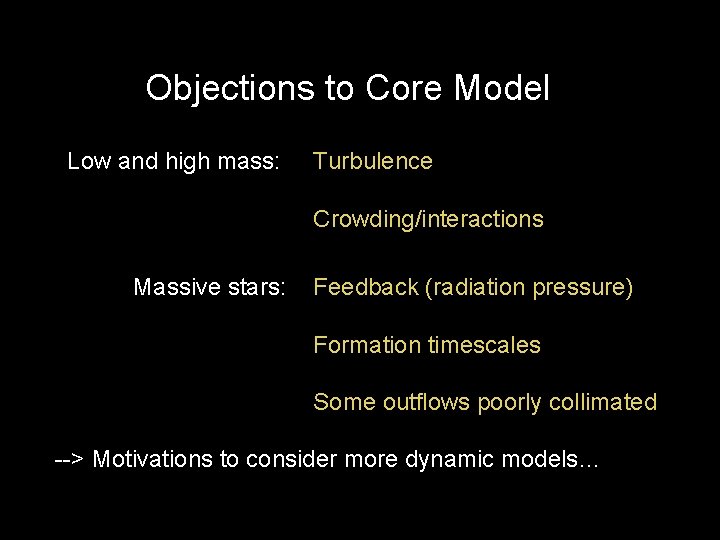
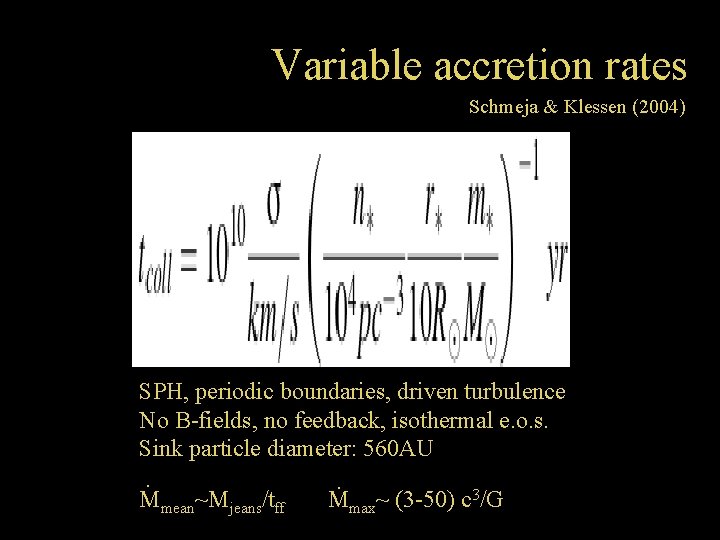
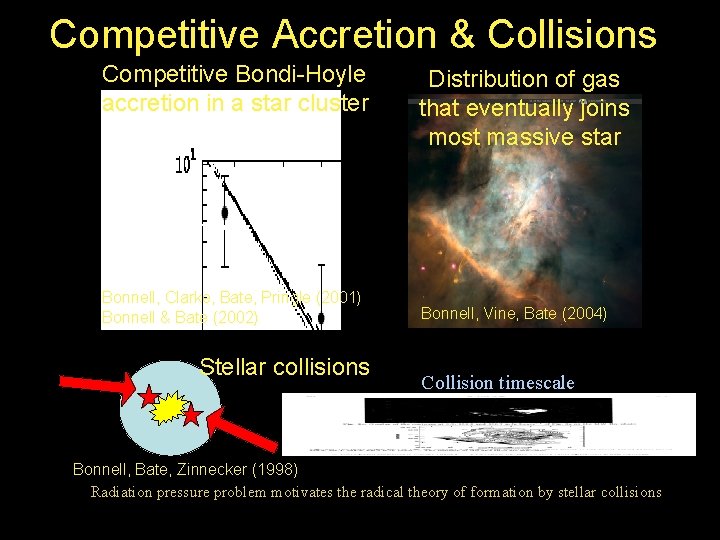
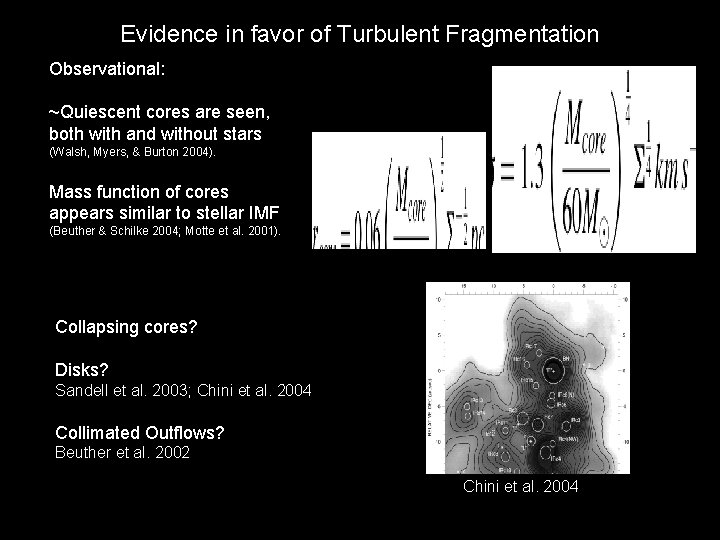
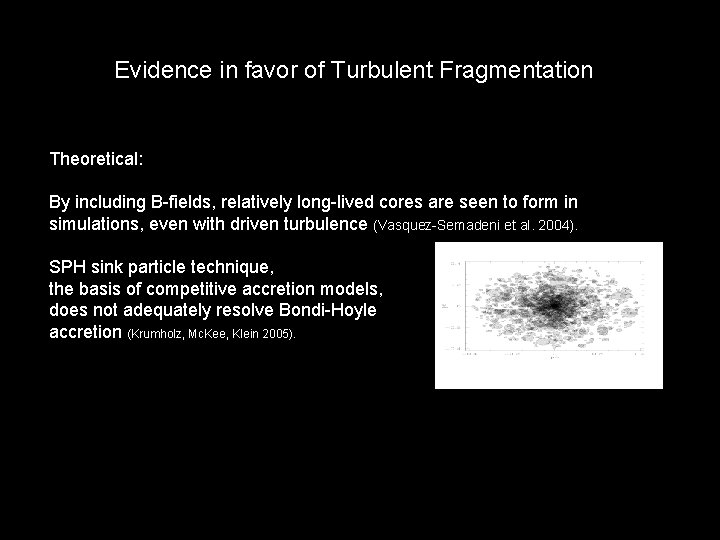
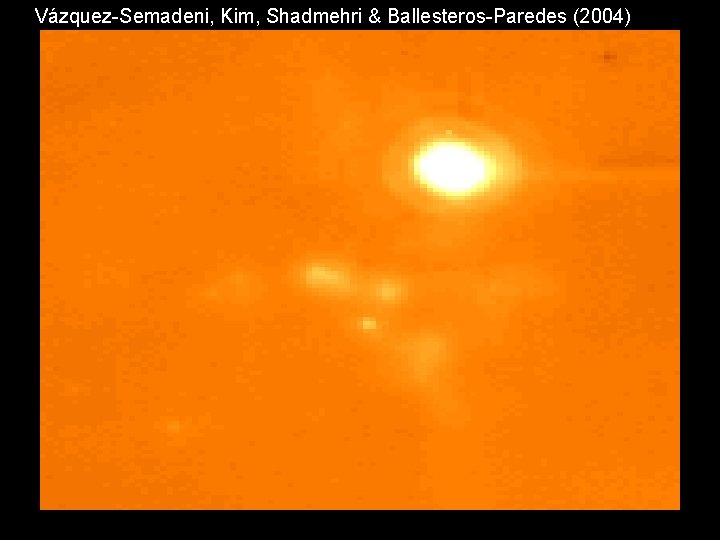
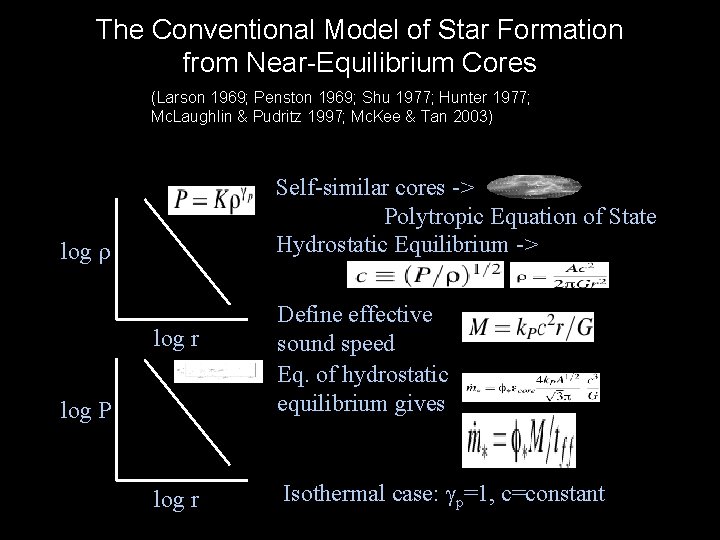
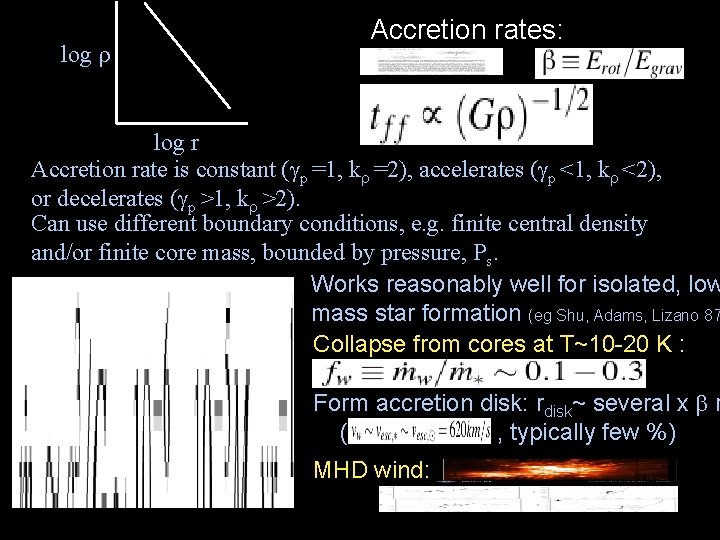
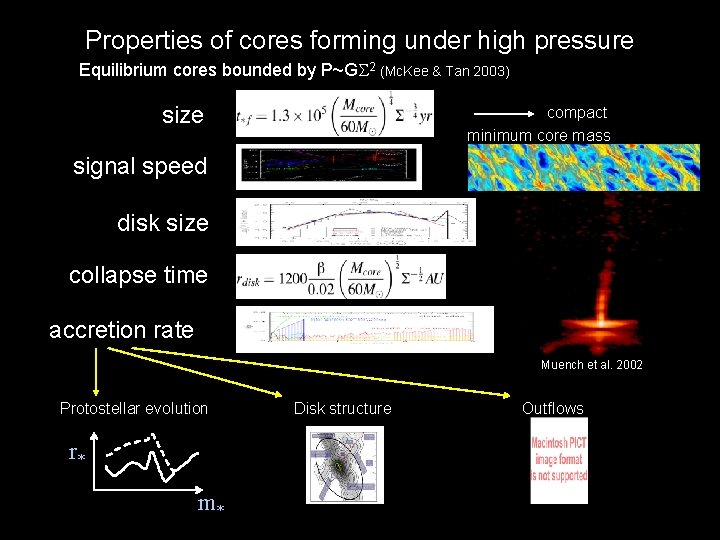
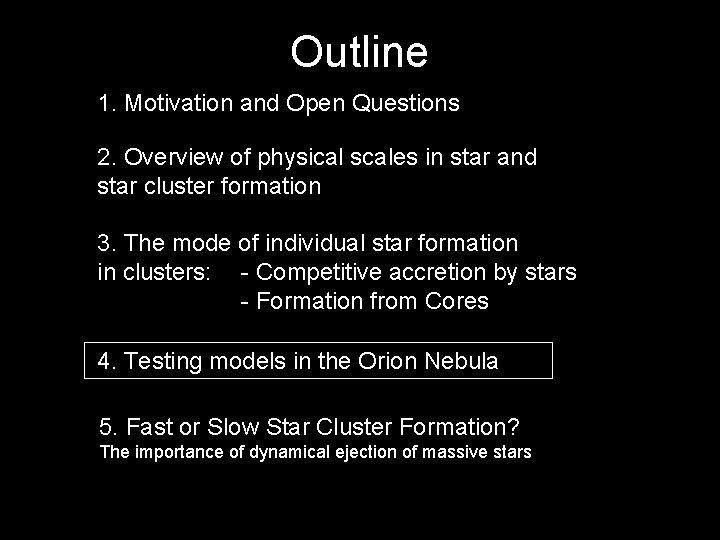
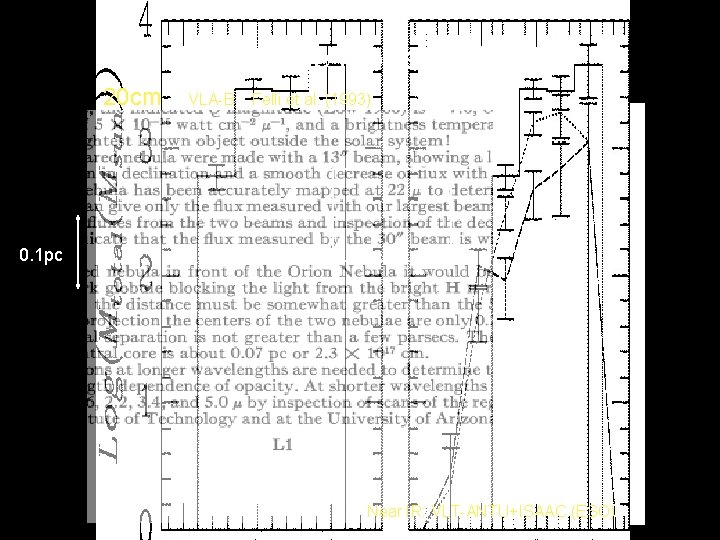
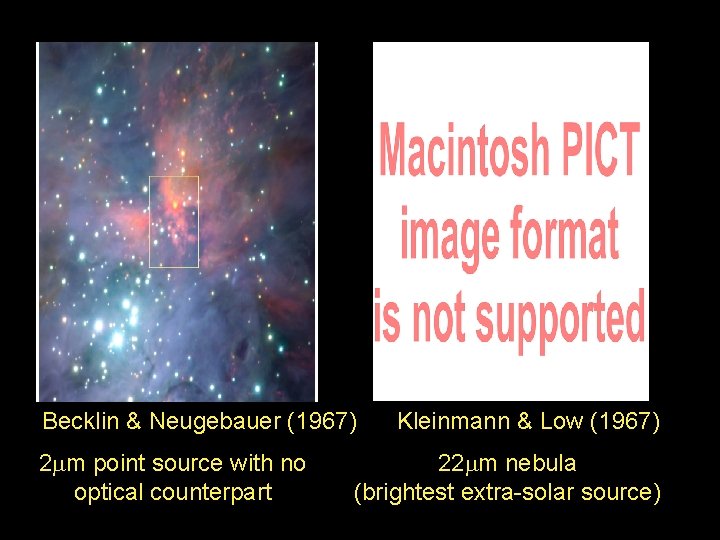
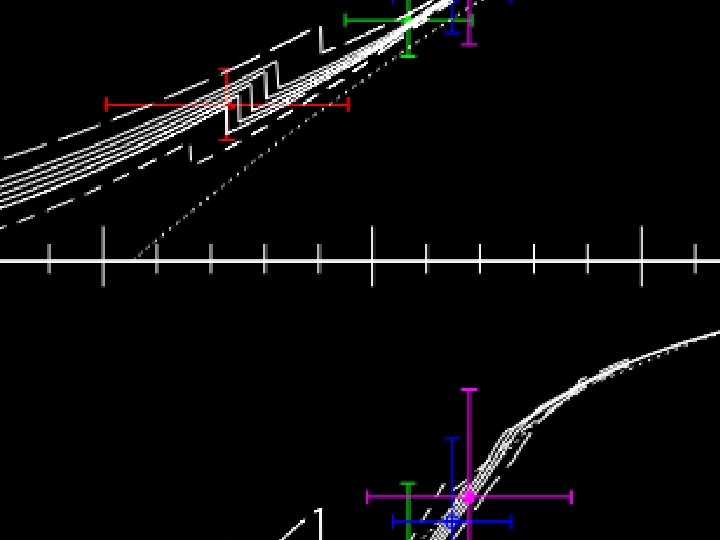
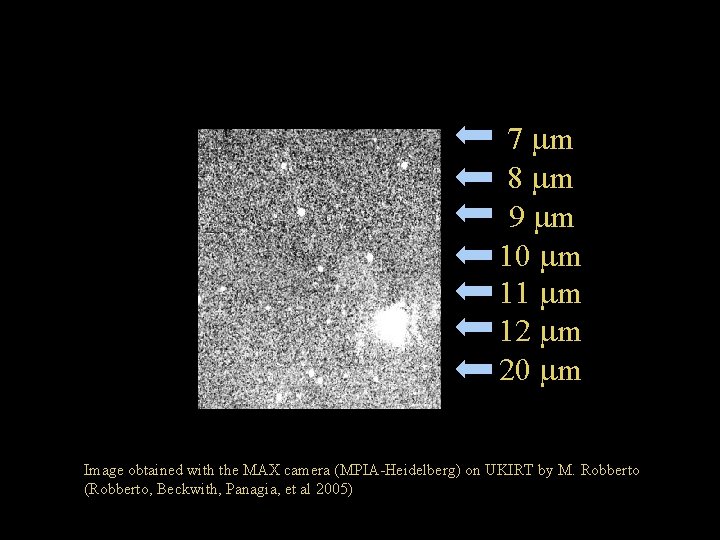
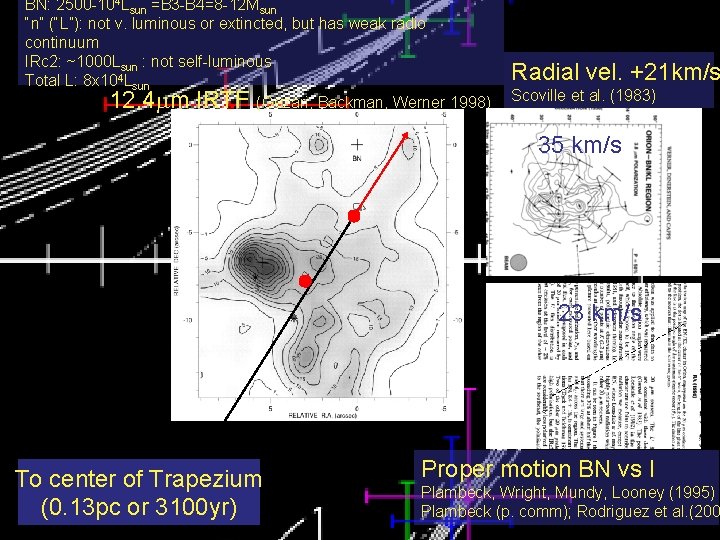
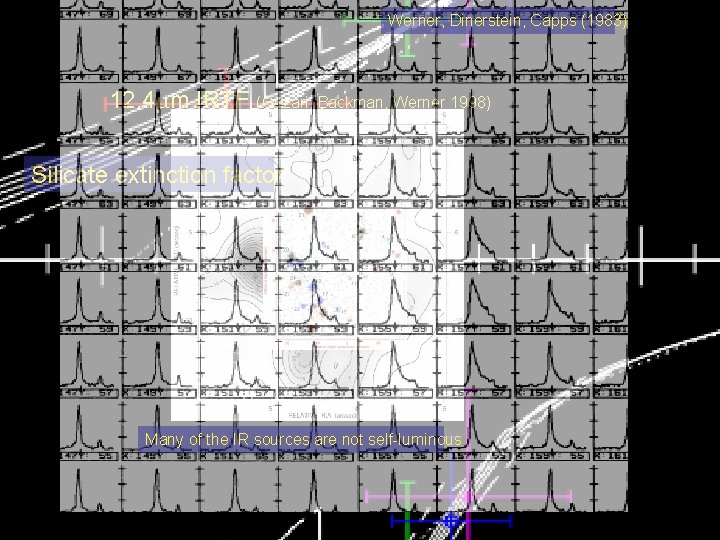
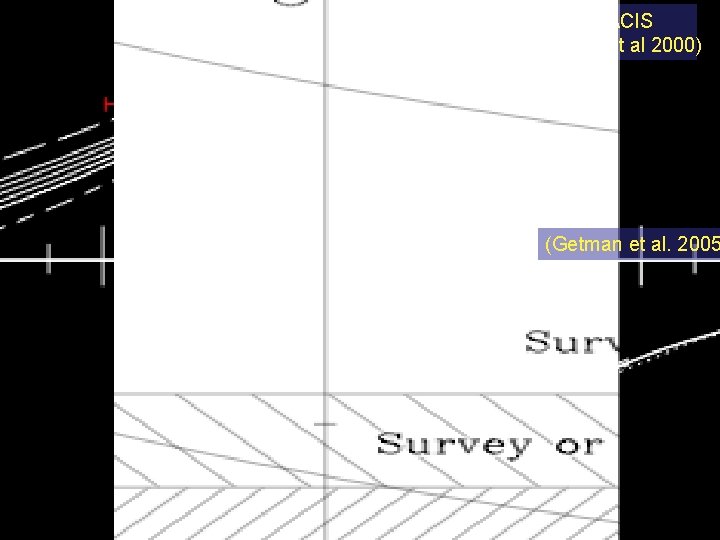
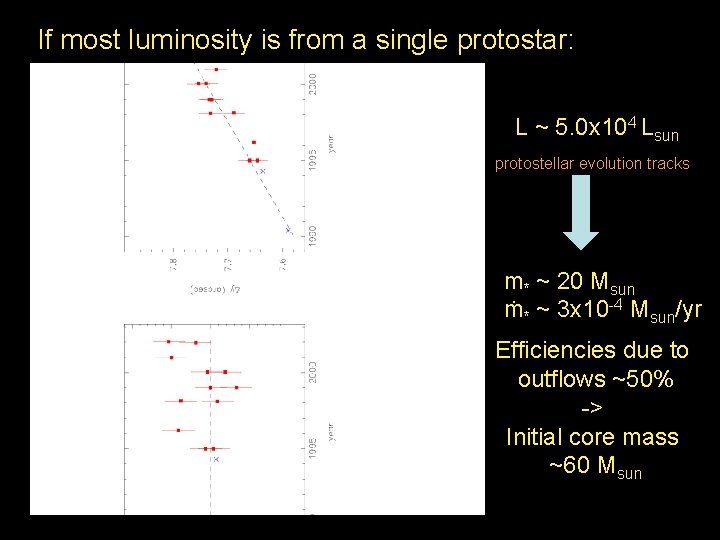
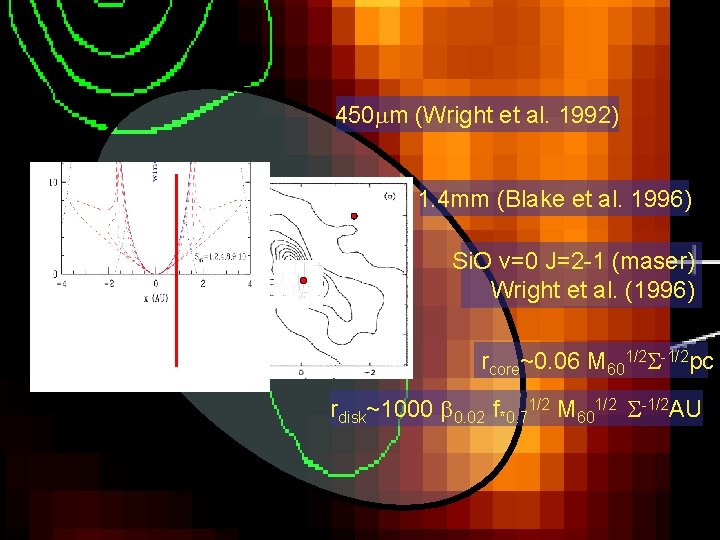
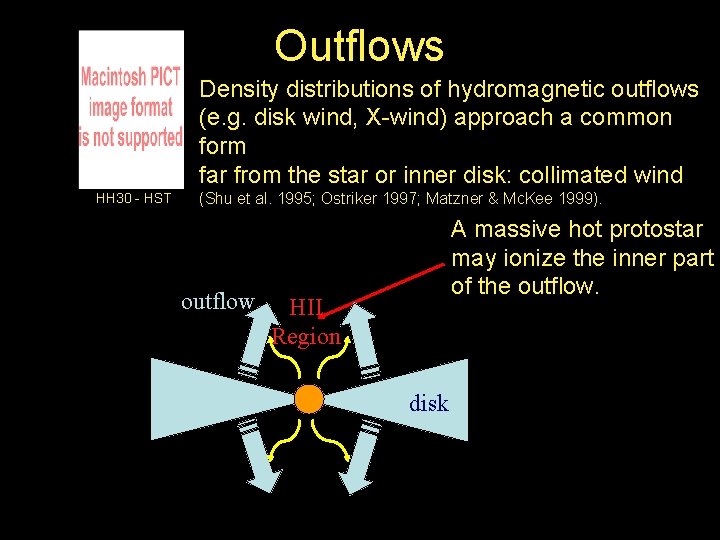
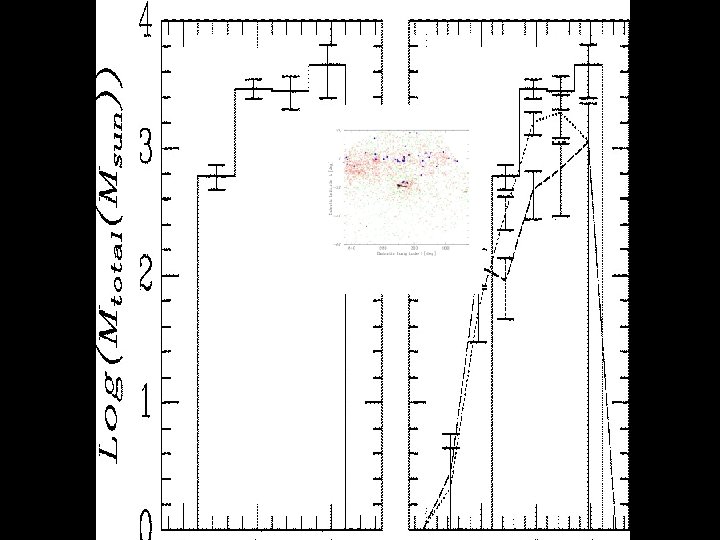
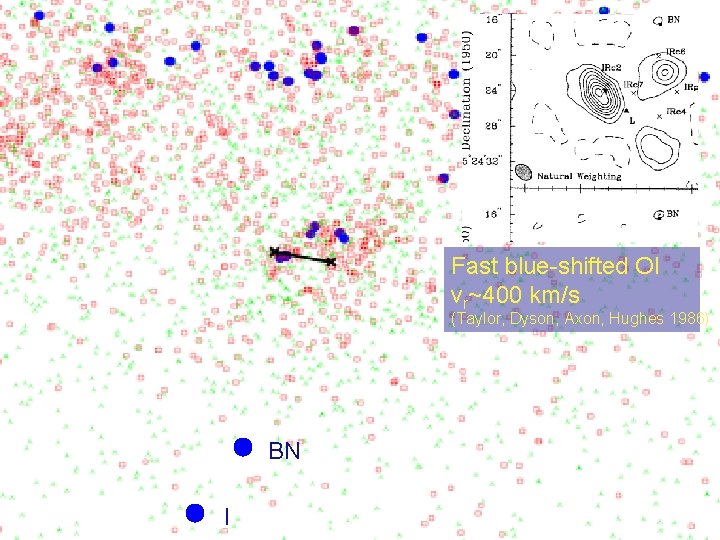
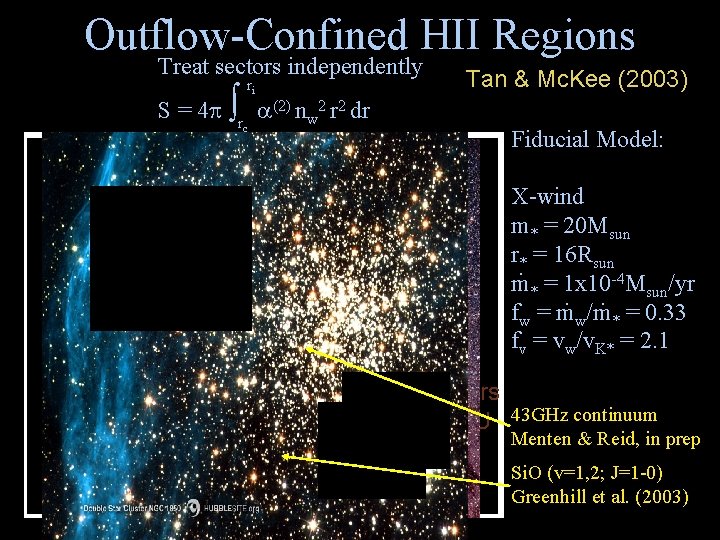
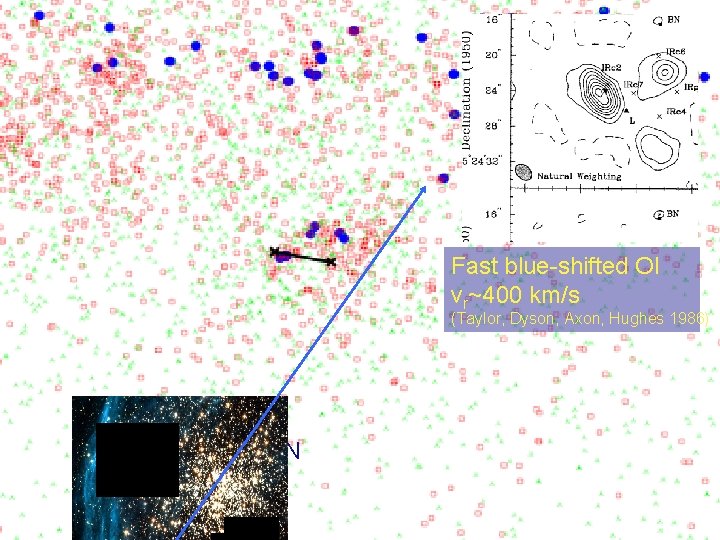
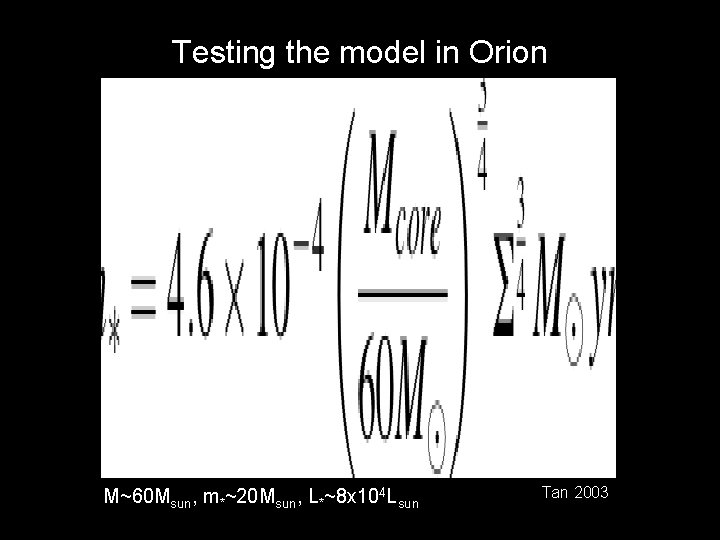
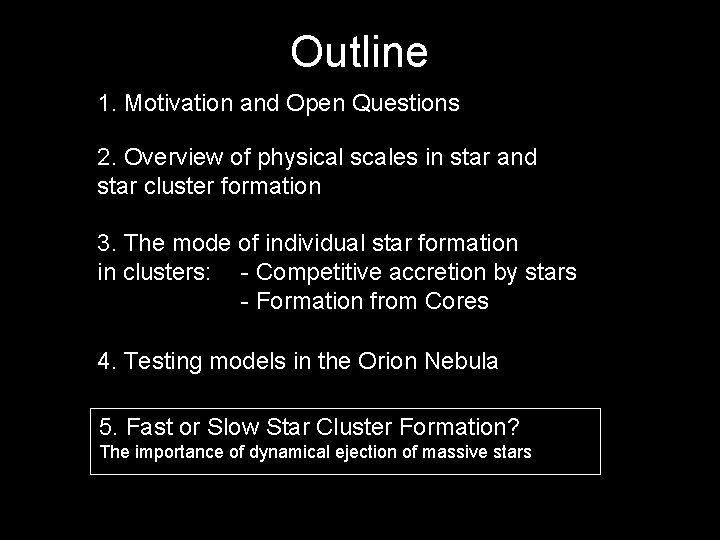
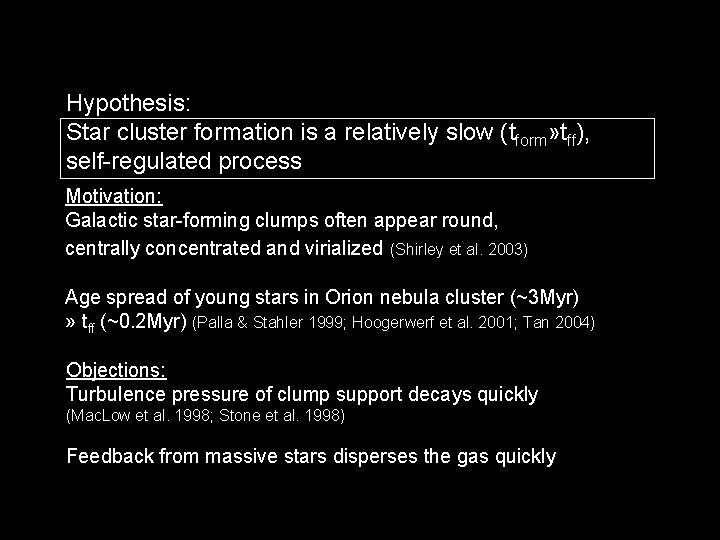
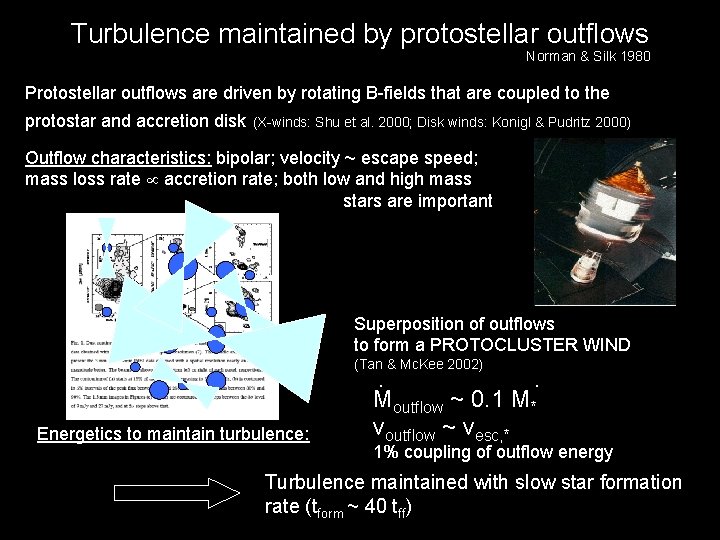
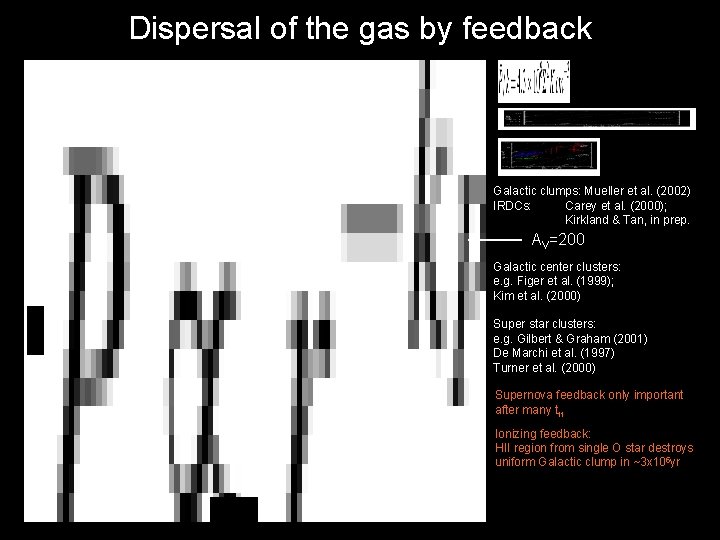
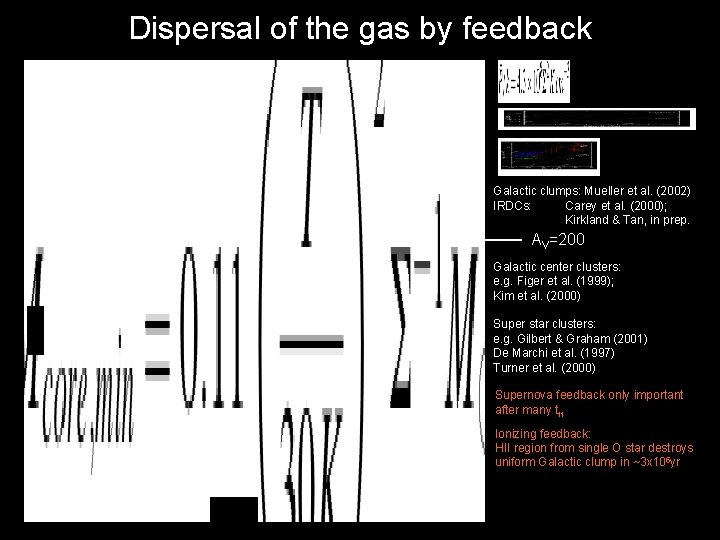
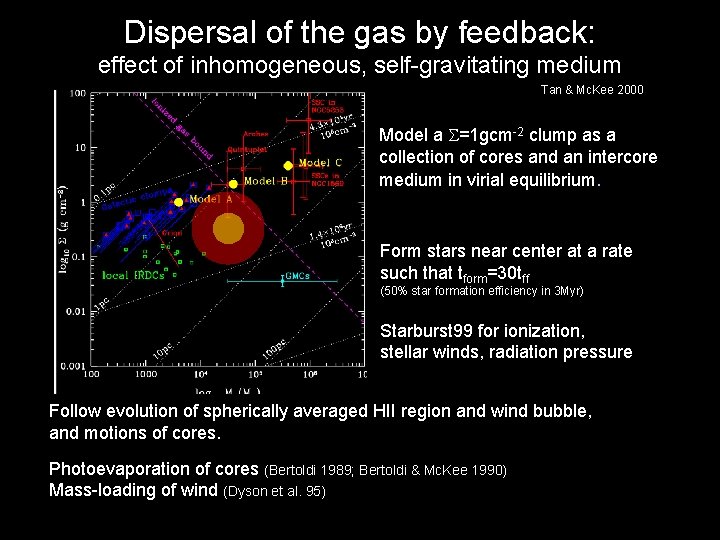
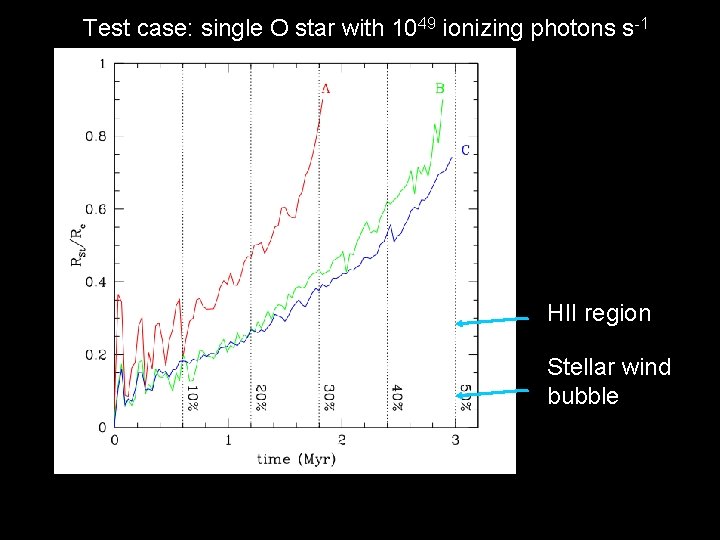
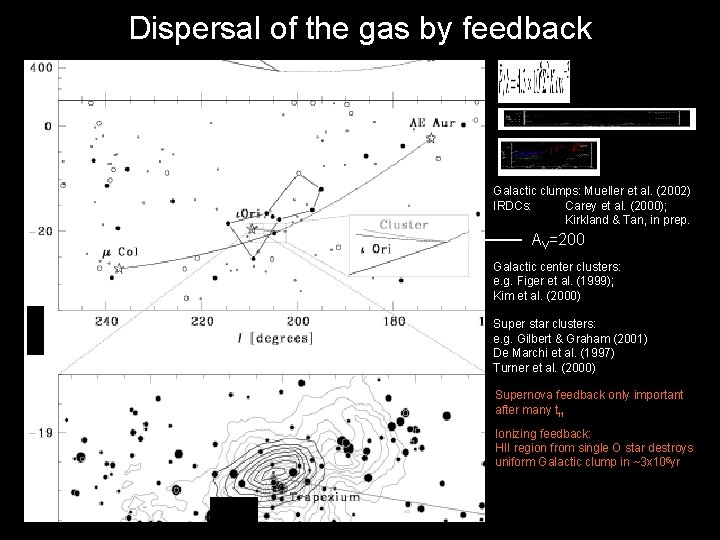
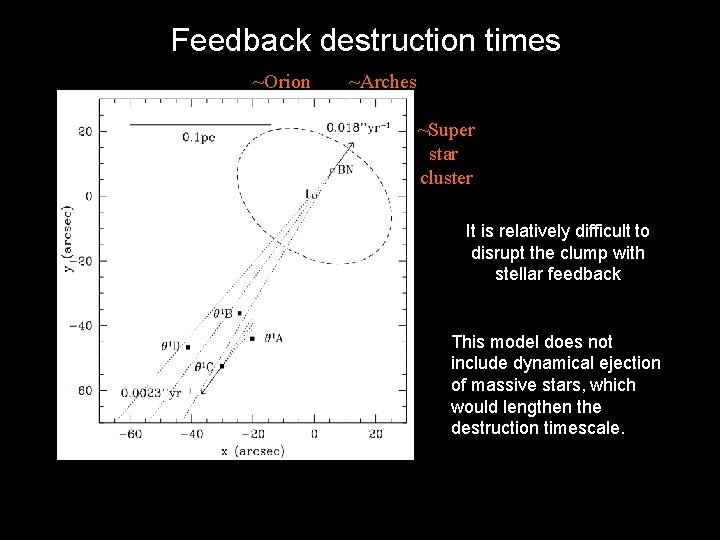
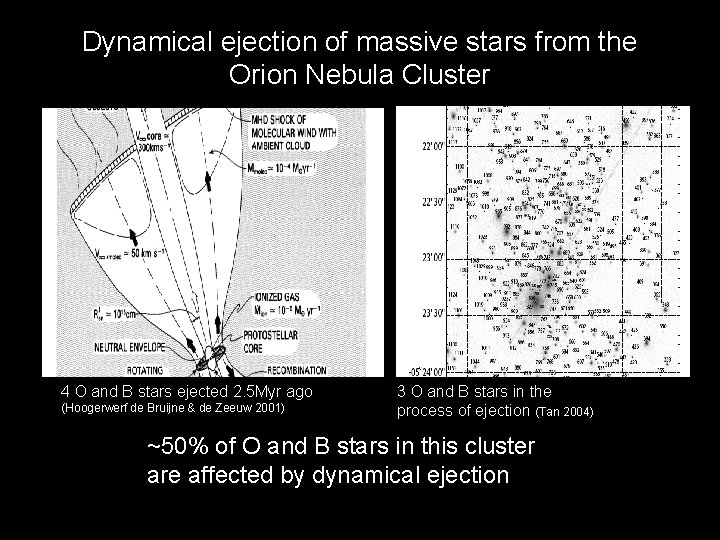
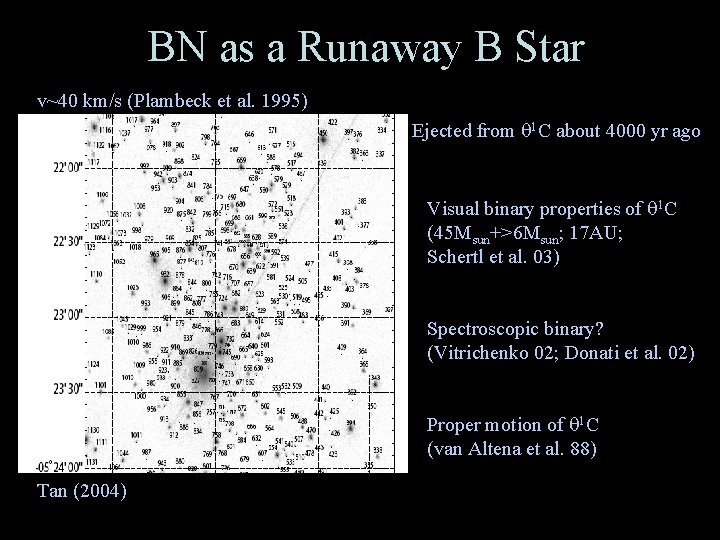
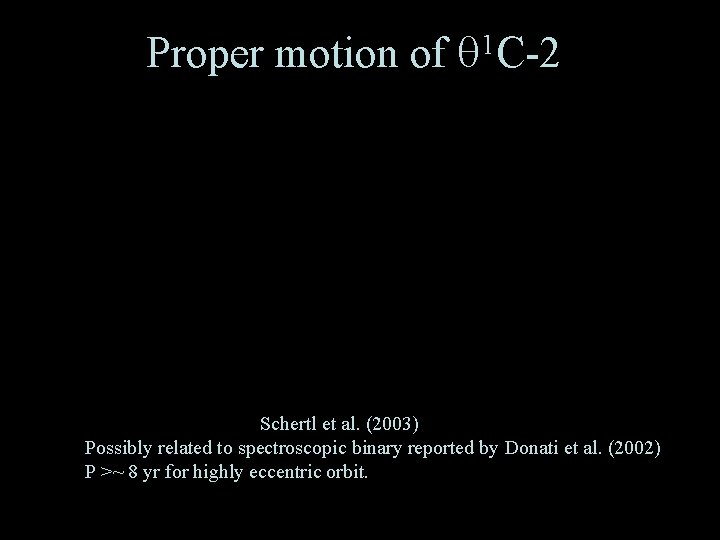
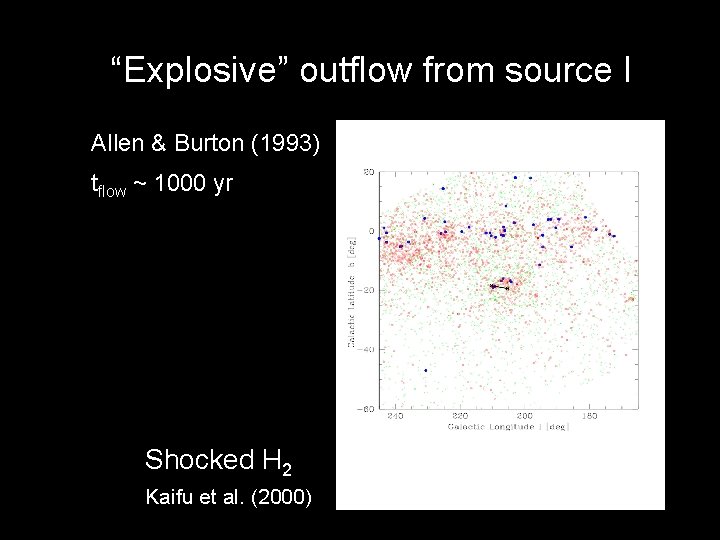
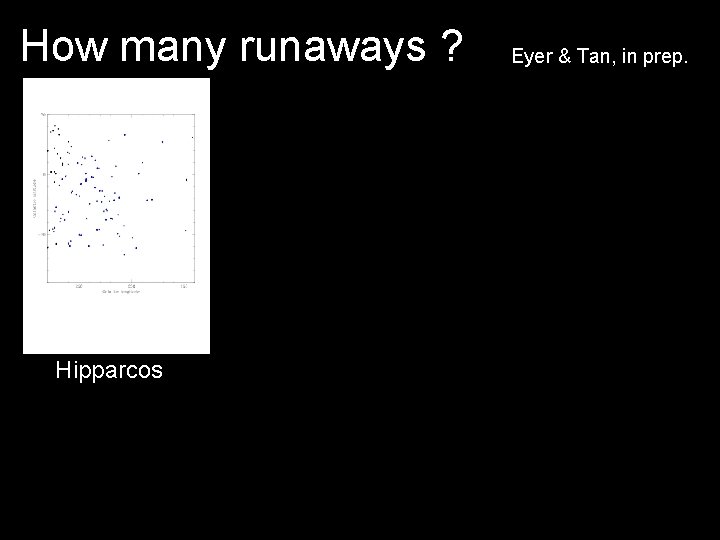
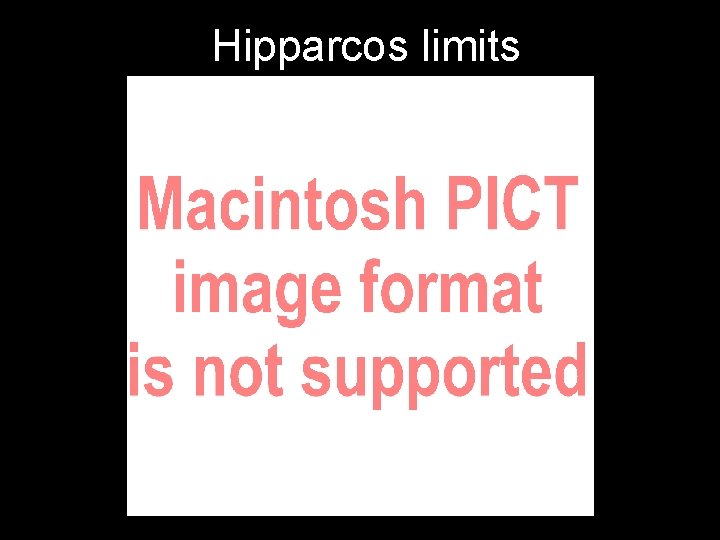
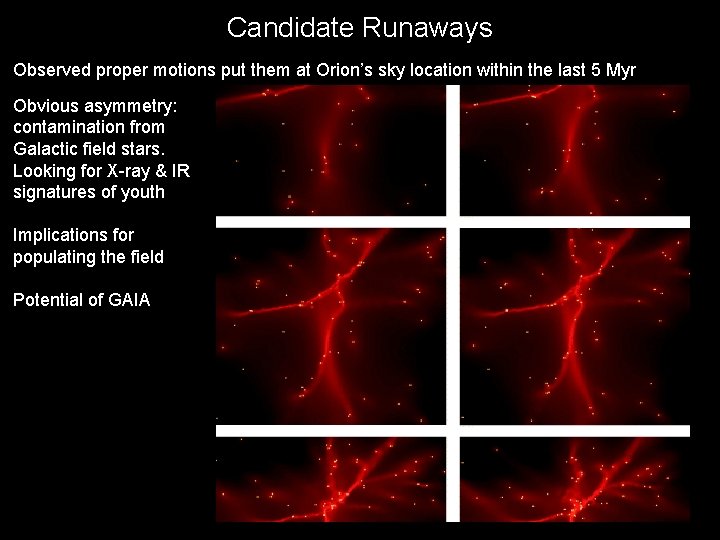
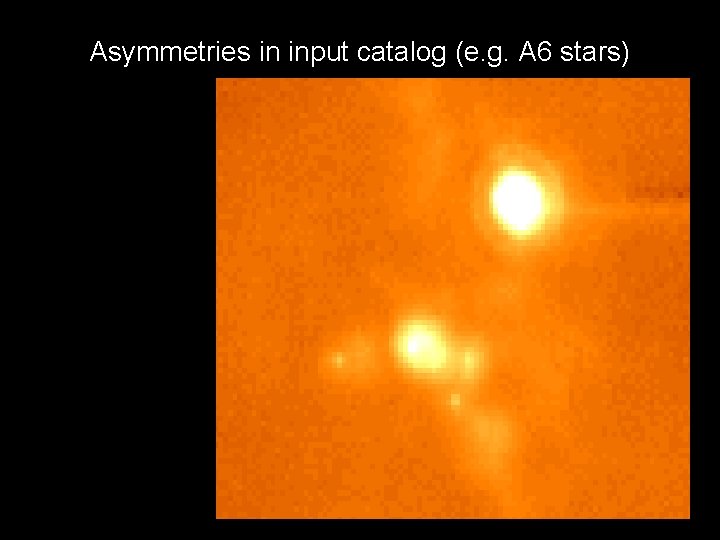
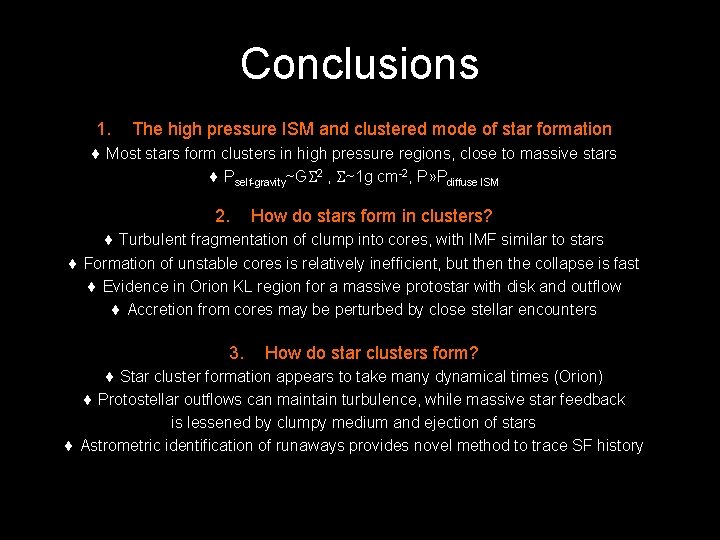
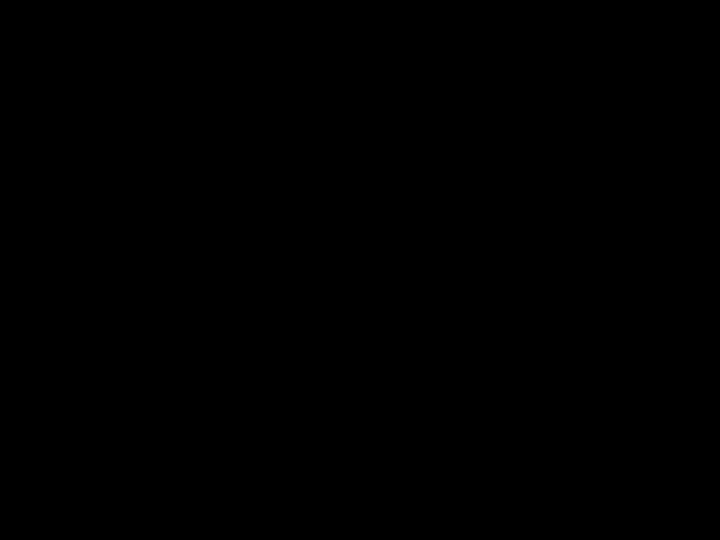
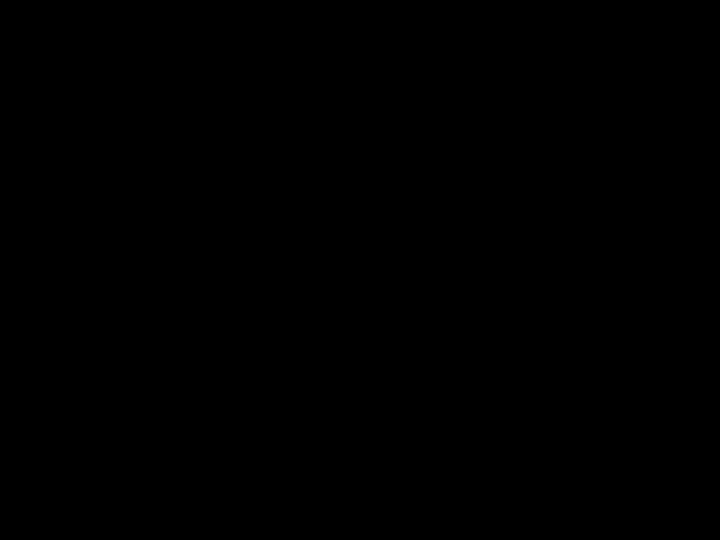
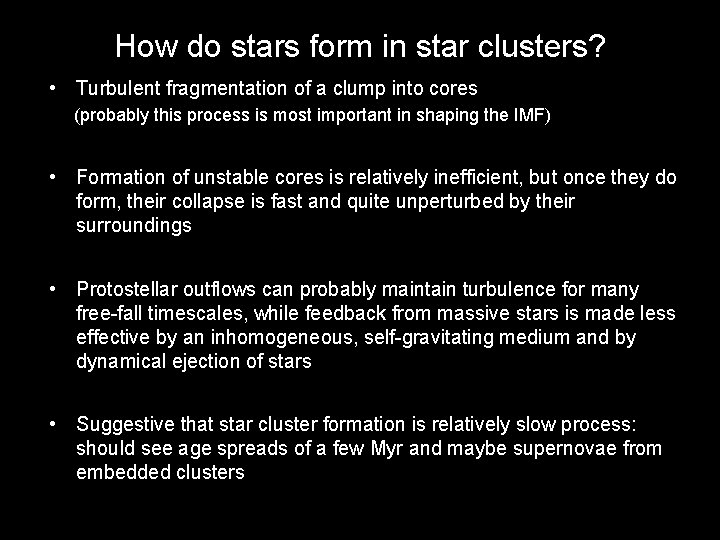
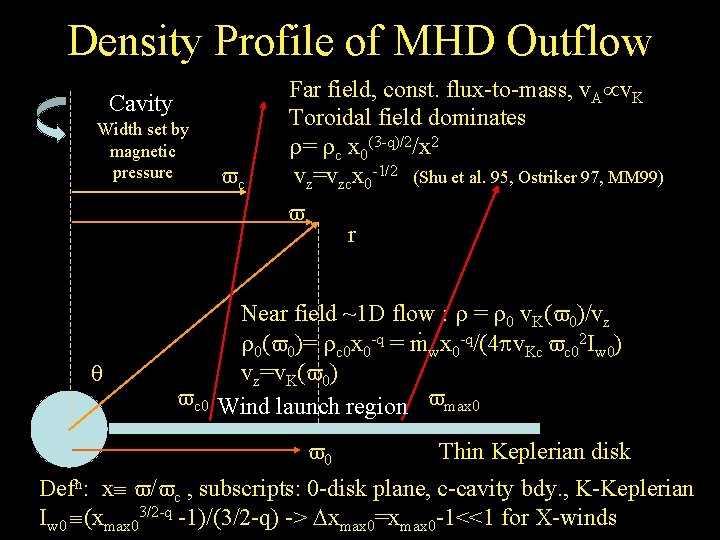
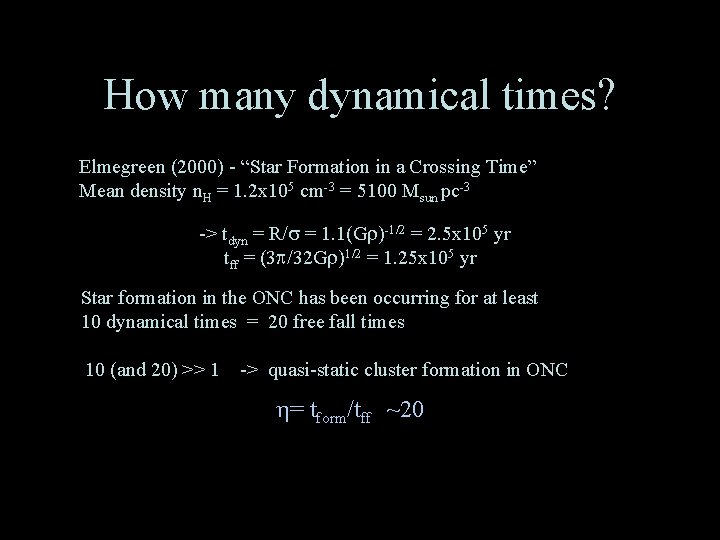
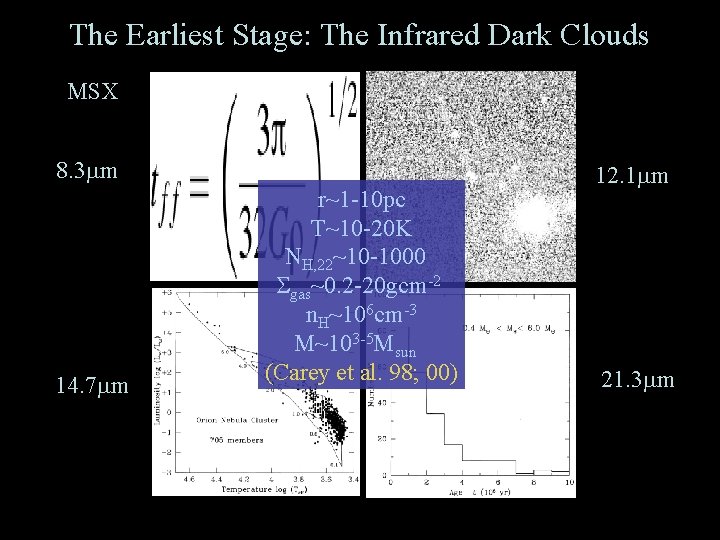
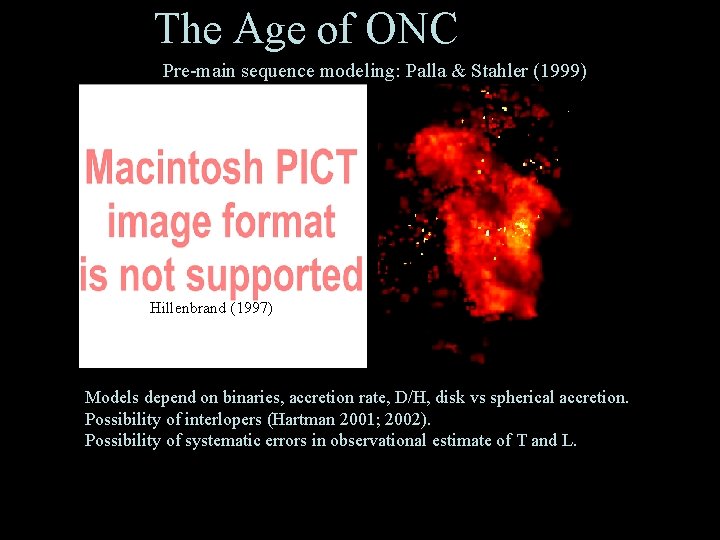
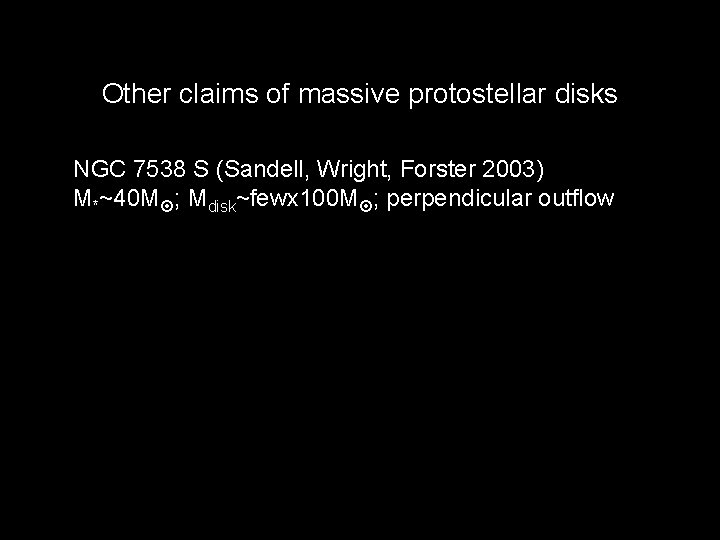
- Slides: 66
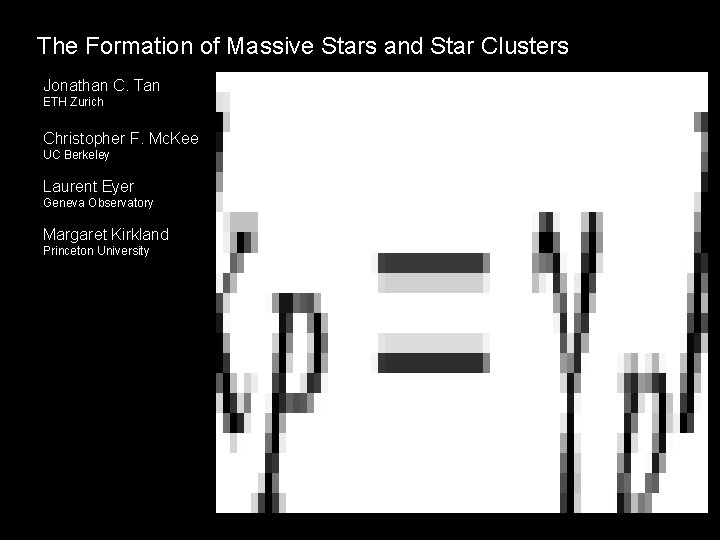
The Formation of Massive Stars and Star Clusters Jonathan C. Tan ETH Zurich Christopher F. Mc. Kee UC Berkeley Laurent Eyer Geneva Observatory Margaret Kirkland Princeton University
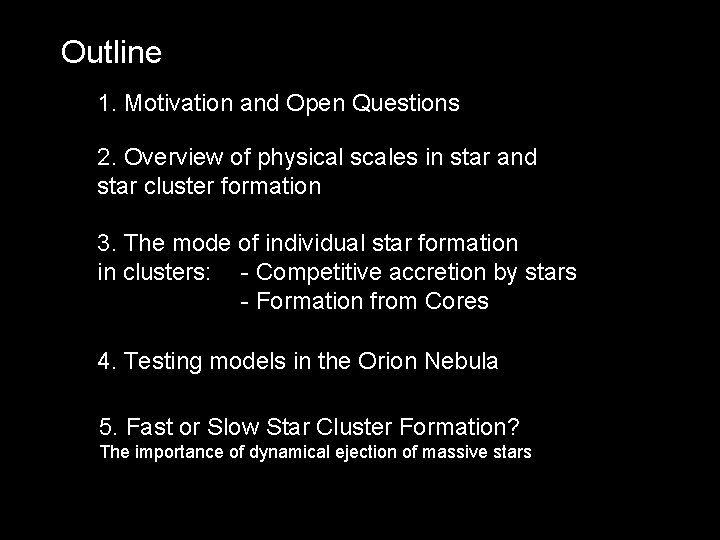
Outline 1. Motivation and Open Questions 2. Overview of physical scales in star and star cluster formation 3. The mode of individual star formation in clusters: - Competitive accretion by stars - Formation from Cores 4. Testing models in the Orion Nebula 5. Fast or Slow Star Cluster Formation? The importance of dynamical ejection of massive stars
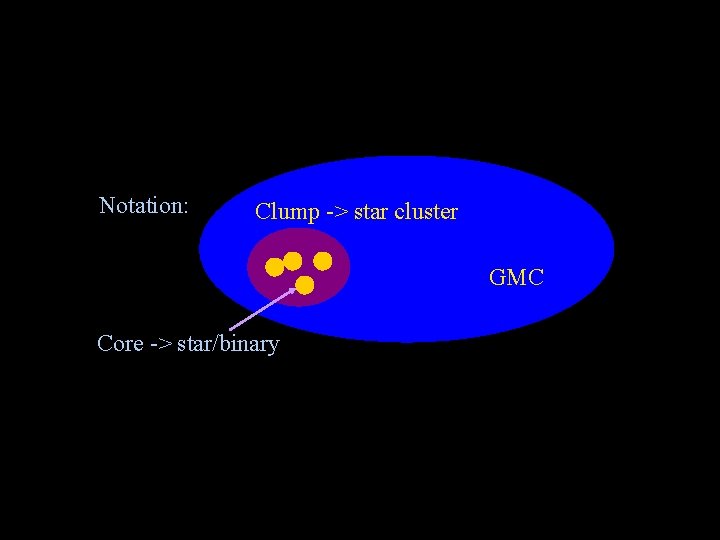
Notation: Clump -> star cluster GMC Core -> star/binary
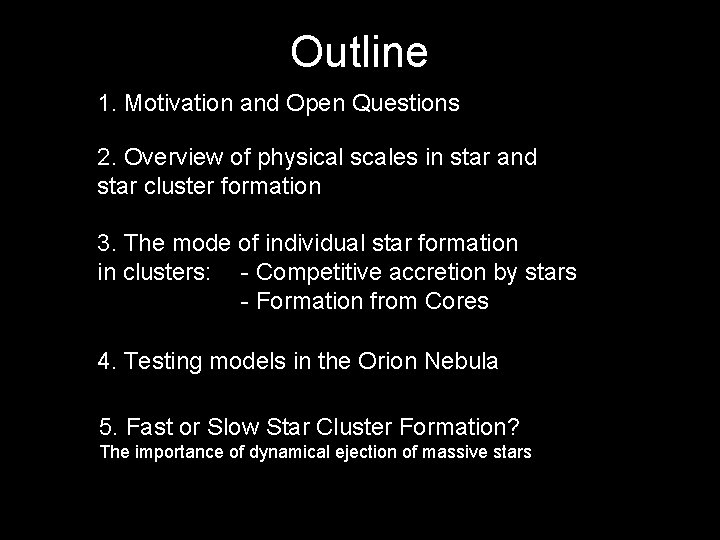
Outline 1. Motivation and Open Questions 2. Overview of physical scales in star and star cluster formation 3. The mode of individual star formation in clusters: - Competitive accretion by stars - Formation from Cores 4. Testing models in the Orion Nebula 5. Fast or Slow Star Cluster Formation? The importance of dynamical ejection of massive stars
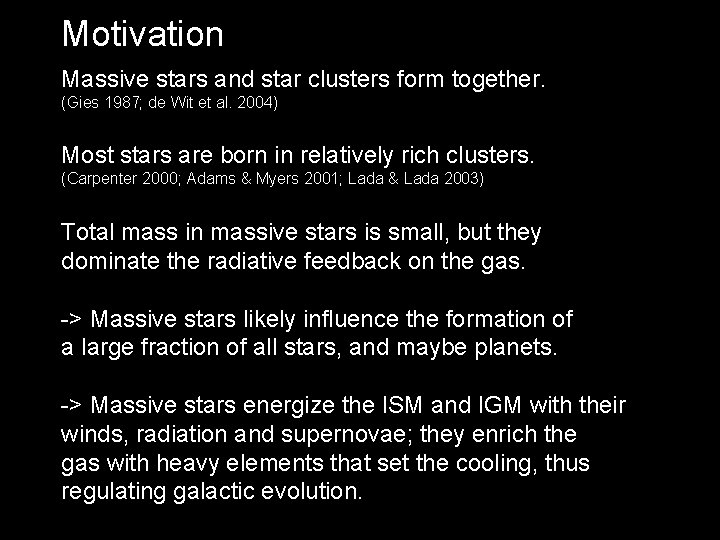
Motivation Massive stars and star clusters form together. (Gies 1987; de Wit et al. 2004) Most stars are born in relatively rich clusters. (Carpenter 2000; Adams & Myers 2001; Lada & Lada 2003) Total mass in massive stars is small, but they dominate the radiative feedback on the gas. -> Massive stars likely influence the formation of a large fraction of all stars, and maybe planets. -> Massive stars energize the ISM and IGM with their winds, radiation and supernovae; they enrich the gas with heavy elements that set the cooling, thus regulating galactic evolution.
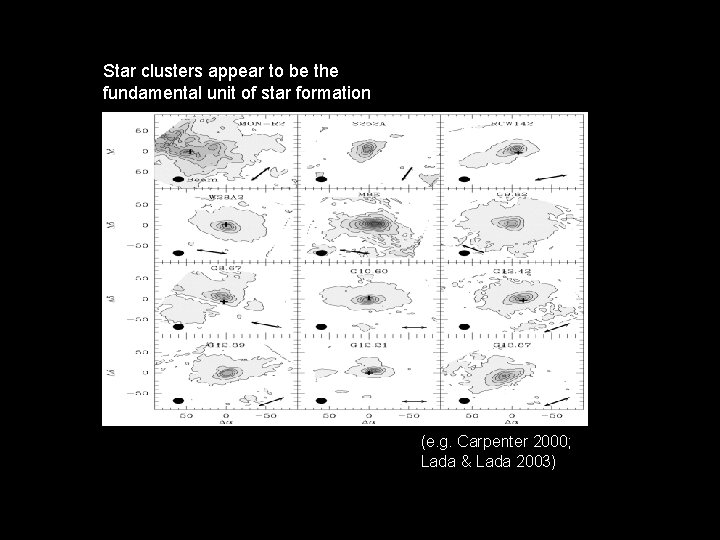
Star clusters appear to be the fundamental unit of star formation (e. g. Carpenter 2000; Lada & Lada 2003)
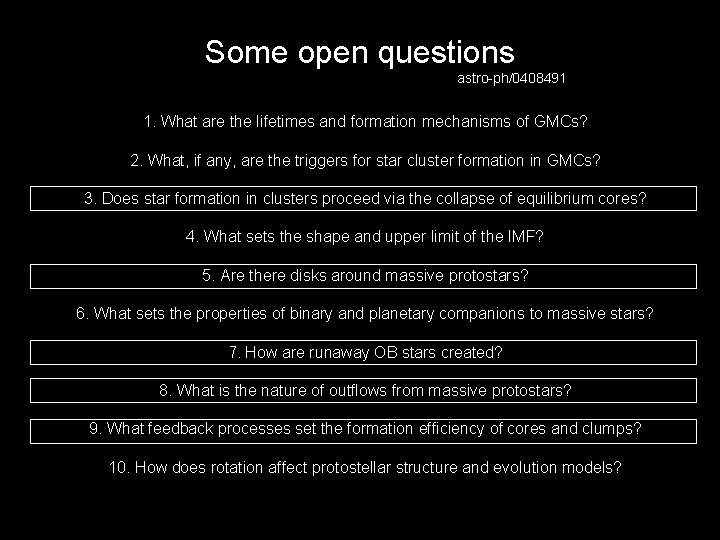
Some open questions astro-ph/0408491 1. What are the lifetimes and formation mechanisms of GMCs? 2. What, if any, are the triggers for star cluster formation in GMCs? 3. Does star formation in clusters proceed via the collapse of equilibrium cores? 4. What sets the shape and upper limit of the IMF? 5. Are there disks around massive protostars? 6. What sets the properties of binary and planetary companions to massive stars? 7. How are runaway OB stars created? 8. What is the nature of outflows from massive protostars? 9. What feedback processes set the formation efficiency of cores and clumps? 10. How does rotation affect protostellar structure and evolution models?
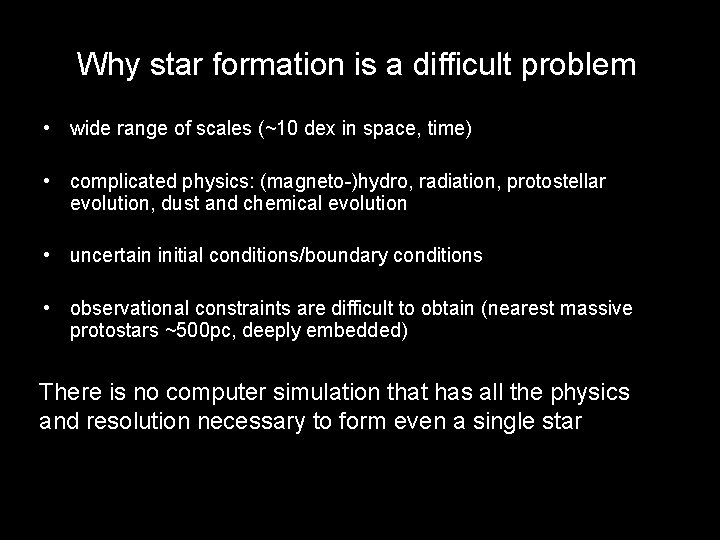
Why star formation is a difficult problem • wide range of scales (~10 dex in space, time) • complicated physics: (magneto-)hydro, radiation, protostellar evolution, dust and chemical evolution • uncertain initial conditions/boundary conditions • observational constraints are difficult to obtain (nearest massive protostars ~500 pc, deeply embedded) There is no computer simulation that has all the physics and resolution necessary to form even a single star
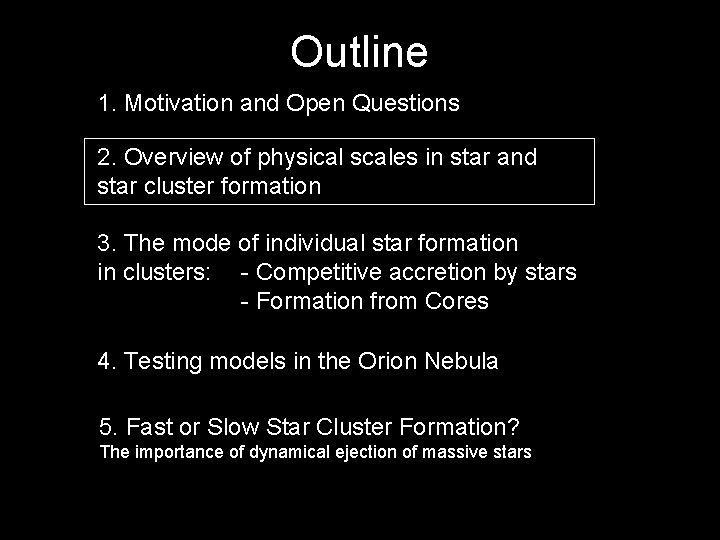
Outline 1. Motivation and Open Questions 2. Overview of physical scales in star and star cluster formation 3. The mode of individual star formation in clusters: - Competitive accretion by stars - Formation from Cores 4. Testing models in the Orion Nebula 5. Fast or Slow Star Cluster Formation? The importance of dynamical ejection of massive stars
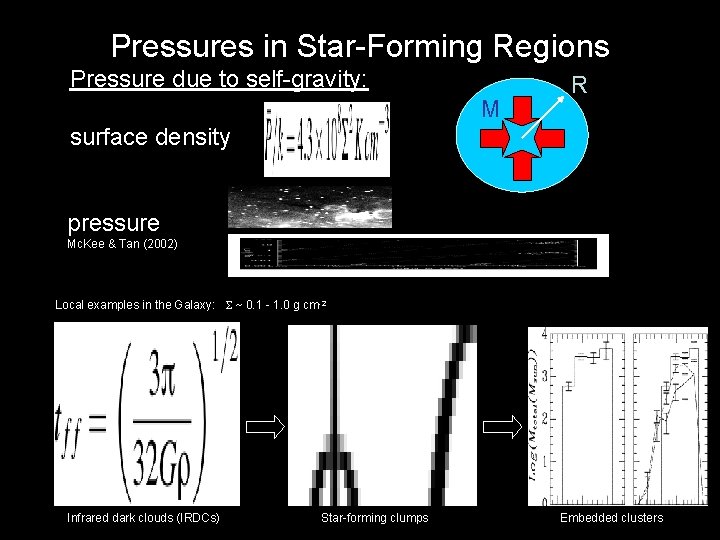
Pressures in Star-Forming Regions Pressure due to self-gravity: M R surface density pressure Mc. Kee & Tan (2002) Local examples in the Galaxy: ~ 0. 1 - 1. 0 g cm-2 Infrared dark clouds (IRDCs) Star-forming clumps Embedded clusters
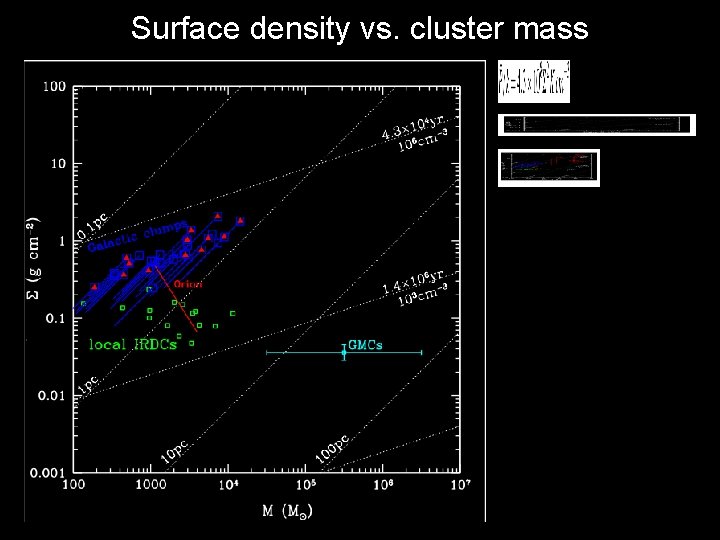
Surface density vs. cluster mass
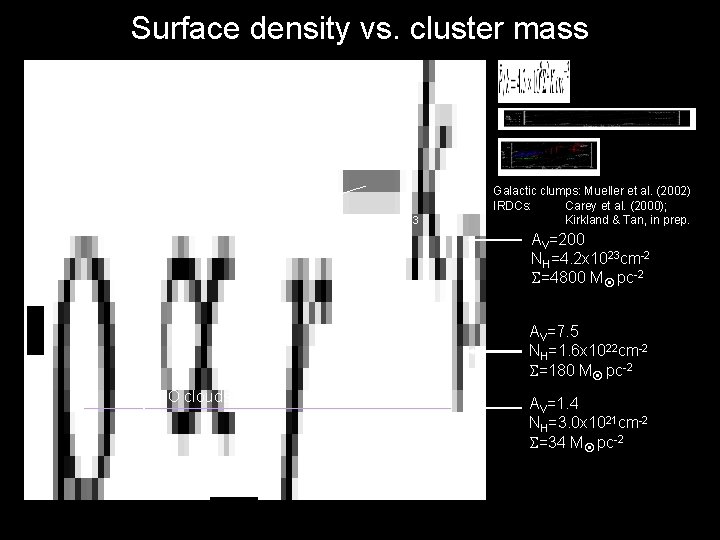
Surface density vs. cluster mass =7000 M pc-3 Galactic clumps: Mueller et al. (2002) IRDCs: Carey et al. (2000); Kirkland & Tan, in prep. AV=200 NH=4. 2 x 1023 cm-2 =4800 M pc-2 AV=7. 5 NH=1. 6 x 1022 cm-2 =180 M pc-2 CO clouds AV=1. 4 NH=3. 0 x 1021 cm-2 =34 M pc-2
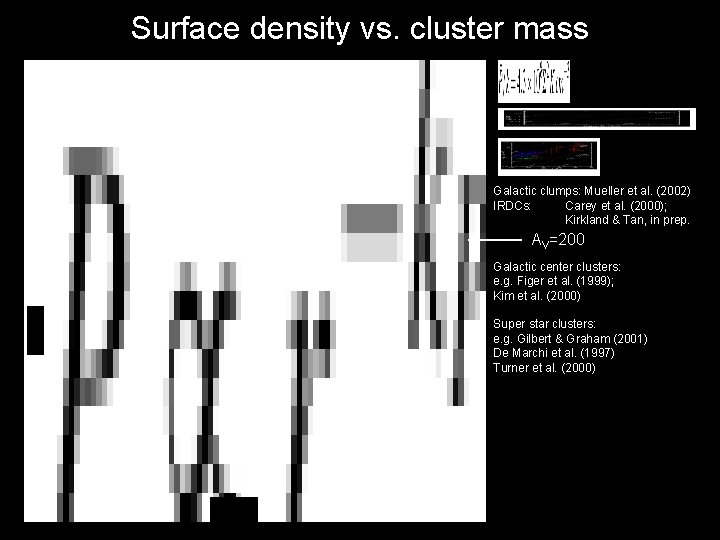
Surface density vs. cluster mass Galactic clumps: Mueller et al. (2002) IRDCs: Carey et al. (2000); Kirkland & Tan, in prep. AV=200 Galactic center clusters: e. g. Figer et al. (1999); Kim et al. (2000) Super star clusters: e. g. Gilbert & Graham (2001) De Marchi et al. (1997) Turner et al. (2000)
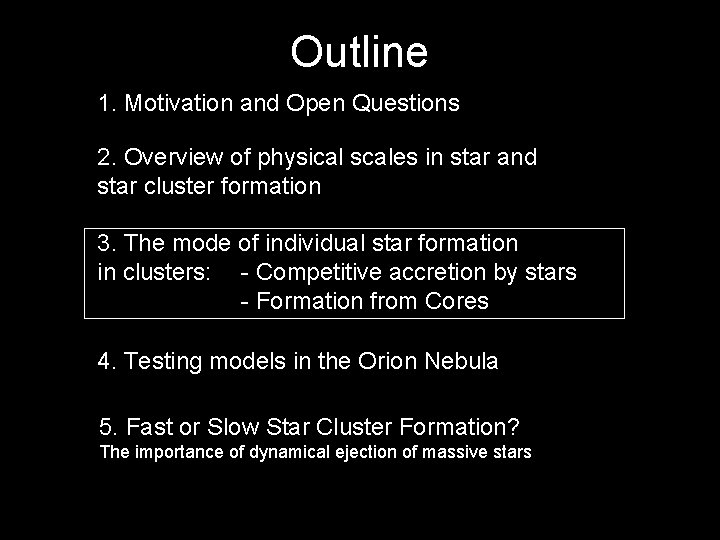
Outline 1. Motivation and Open Questions 2. Overview of physical scales in star and star cluster formation 3. The mode of individual star formation in clusters: - Competitive accretion by stars - Formation from Cores 4. Testing models in the Orion Nebula 5. Fast or Slow Star Cluster Formation? The importance of dynamical ejection of massive stars
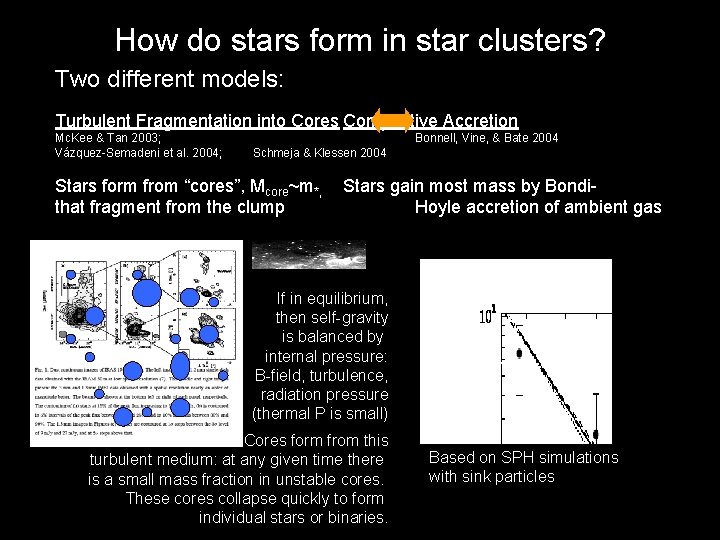
How do stars form in star clusters? Two different models: Turbulent Fragmentation into Cores Competitive Accretion Mc. Kee & Tan 2003; Vázquez-Semadeni et al. 2004; Bonnell, Vine, & Bate 2004 Schmeja & Klessen 2004 Stars form from “cores”, Mcore~m*, that fragment from the clump Stars gain most mass by Bondi. Hoyle accretion of ambient gas If in equilibrium, then self-gravity is balanced by internal pressure: B-field, turbulence, radiation pressure (thermal P is small) Cores form from this turbulent medium: at any given time there is a small mass fraction in unstable cores. These cores collapse quickly to form individual stars or binaries. Based on SPH simulations with sink particles
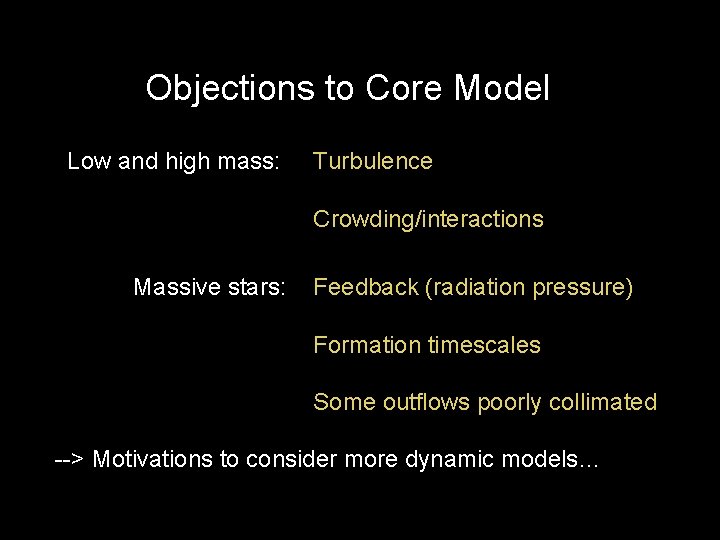
Objections to Core Model Low and high mass: Turbulence Crowding/interactions Massive stars: Feedback (radiation pressure) Formation timescales Some outflows poorly collimated --> Motivations to consider more dynamic models…
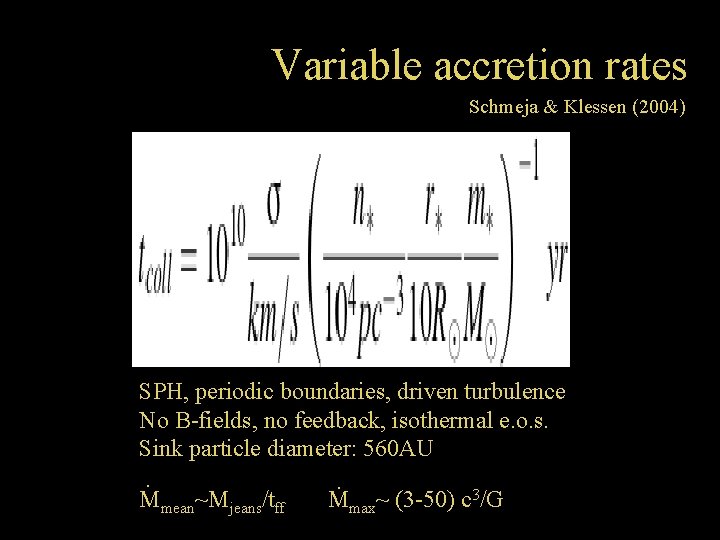
Variable accretion rates Schmeja & Klessen (2004) SPH, periodic boundaries, driven turbulence No B-fields, no feedback, isothermal e. o. s. Sink particle diameter: 560 AU. . Mmean~Mjeans/tff Mmax~ (3 -50) c 3/G
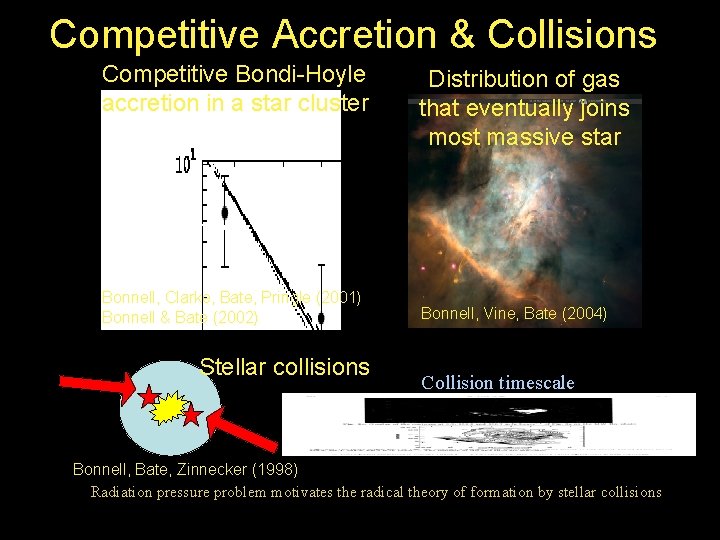
Competitive Accretion & Collisions Competitive Bondi-Hoyle accretion in a star cluster Bonnell, Clarke, Bate, Pringle (2001) Bonnell & Bate (2002) Stellar collisions Distribution of gas that eventually joins most massive star Bonnell, Vine, Bate (2004) Collision timescale Bonnell, Bate, Zinnecker (1998) Radiation pressure problem motivates the radical theory of formation by stellar collisions
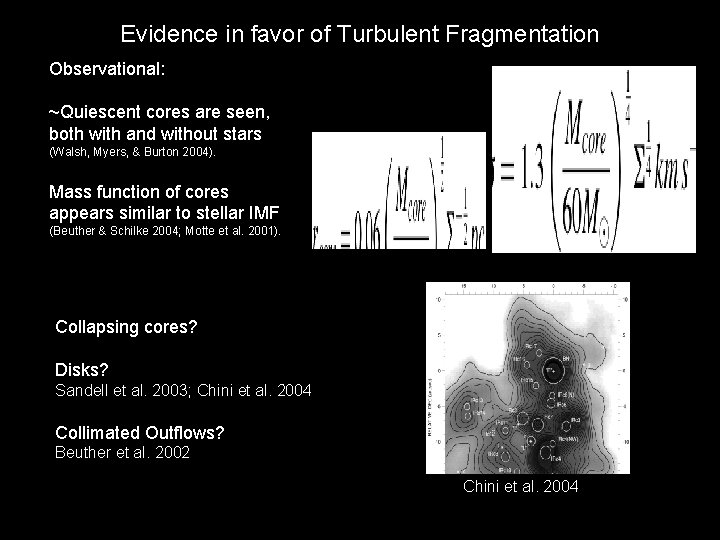
Evidence in favor of Turbulent Fragmentation Observational: ~Quiescent cores are seen, both with and without stars (Walsh, Myers, & Burton 2004). Mass function of cores appears similar to stellar IMF (Beuther & Schilke 2004; Motte et al. 2001). Collapsing cores? Disks? Sandell et al. 2003; Chini et al. 2004 Collimated Outflows? Beuther et al. 2002 Chini et al. 2004
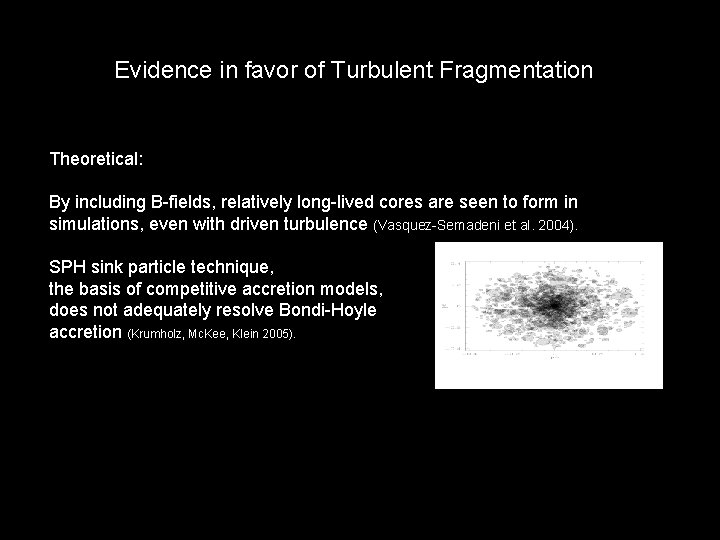
Evidence in favor of Turbulent Fragmentation Theoretical: By including B-fields, relatively long-lived cores are seen to form in simulations, even with driven turbulence (Vasquez-Semadeni et al. 2004). SPH sink particle technique, the basis of competitive accretion models, does not adequately resolve Bondi-Hoyle accretion (Krumholz, Mc. Kee, Klein 2005).
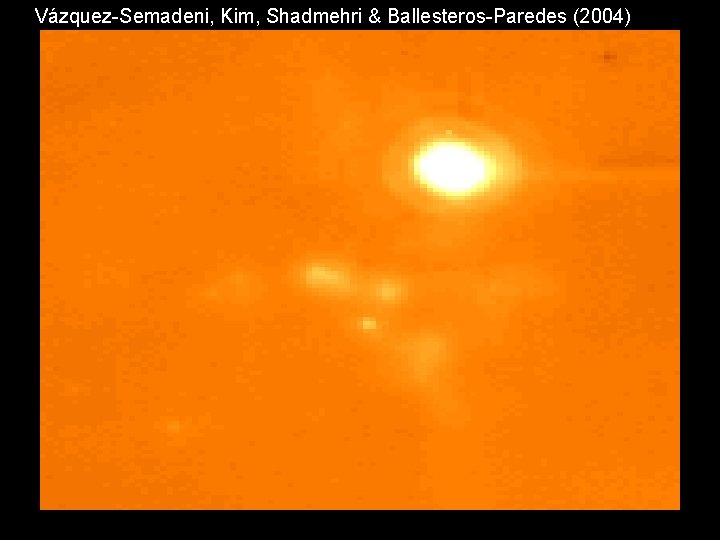
Vázquez-Semadeni, Kim, Shadmehri & Ballesteros-Paredes (2004)
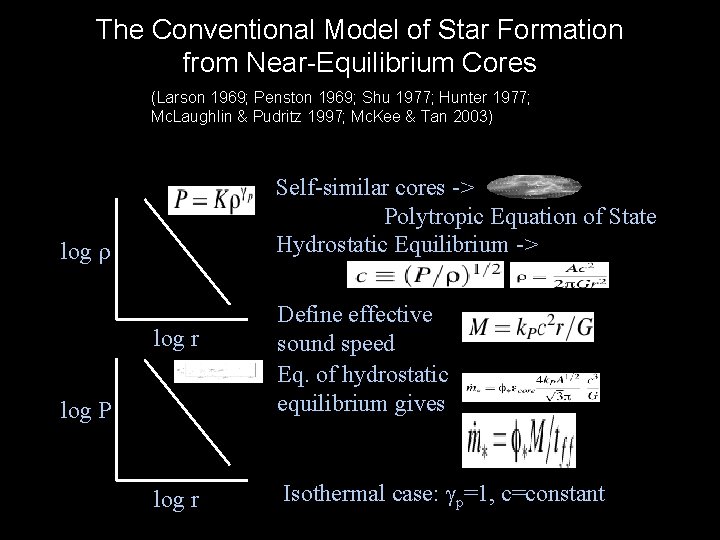
The Conventional Model of Star Formation from Near-Equilibrium Cores (Larson 1969; Penston 1969; Shu 1977; Hunter 1977; Mc. Laughlin & Pudritz 1997; Mc. Kee & Tan 2003) log Self-similar cores -> Polytropic Equation of State Hydrostatic Equilibrium -> log P Define effective sound speed Eq. of hydrostatic equilibrium gives log r Isothermal case: p=1, c=constant
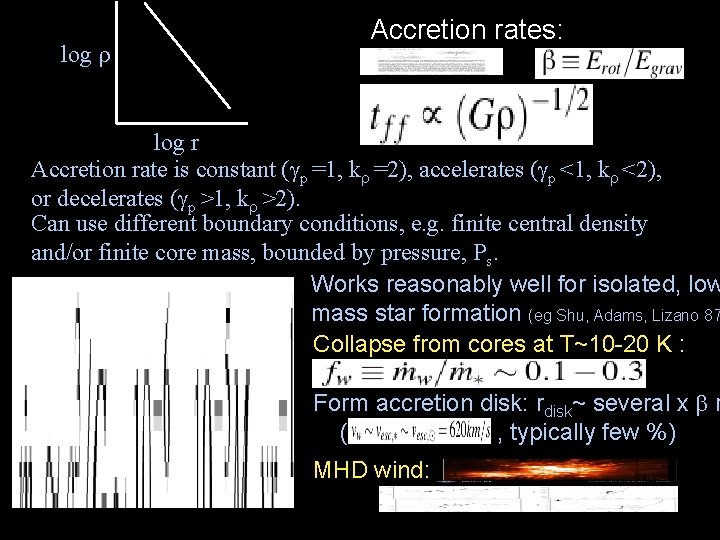
log Accretion rates: log r Accretion rate is constant ( p =1, k =2), accelerates ( p <1, k <2), or decelerates ( p >1, k >2). Can use different boundary conditions, e. g. finite central density and/or finite core mass, bounded by pressure, Ps. Works reasonably well for isolated, low mass star formation (eg Shu, Adams, Lizano 87 Collapse from cores at T~10 -20 K : Form accretion disk: rdisk~ several x r ( , typically few %) MHD wind:
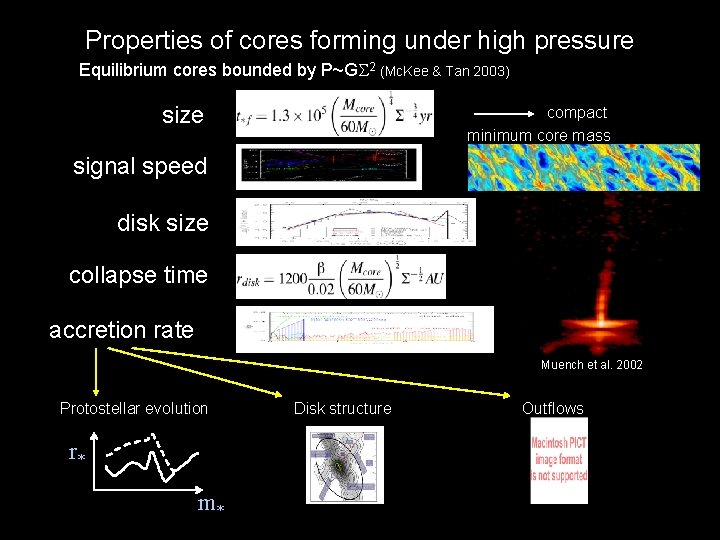
Properties of cores forming under high pressure Equilibrium cores bounded by P~G 2 (Mc. Kee & Tan 2003) size compact minimum core mass signal speed turbulent disk size collapse time accretion rate Muench et al. 2002 Protostellar evolution r* m* Disk structure Outflows
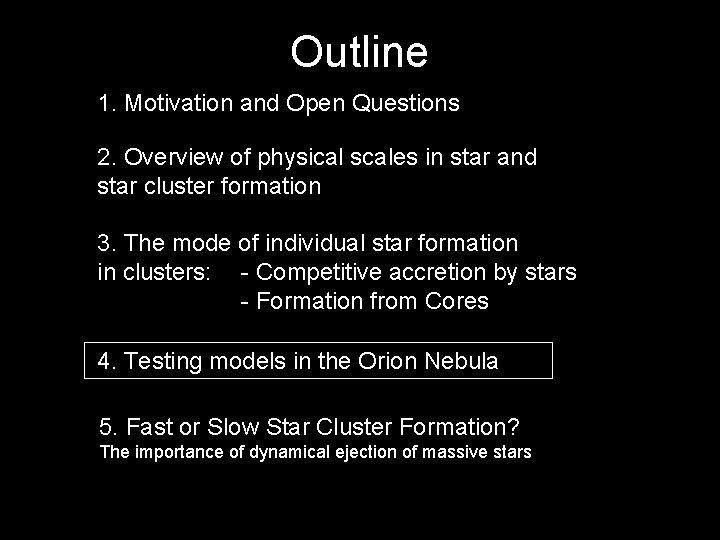
Outline 1. Motivation and Open Questions 2. Overview of physical scales in star and star cluster formation 3. The mode of individual star formation in clusters: - Competitive accretion by stars - Formation from Cores 4. Testing models in the Orion Nebula 5. Fast or Slow Star Cluster Formation? The importance of dynamical ejection of massive stars
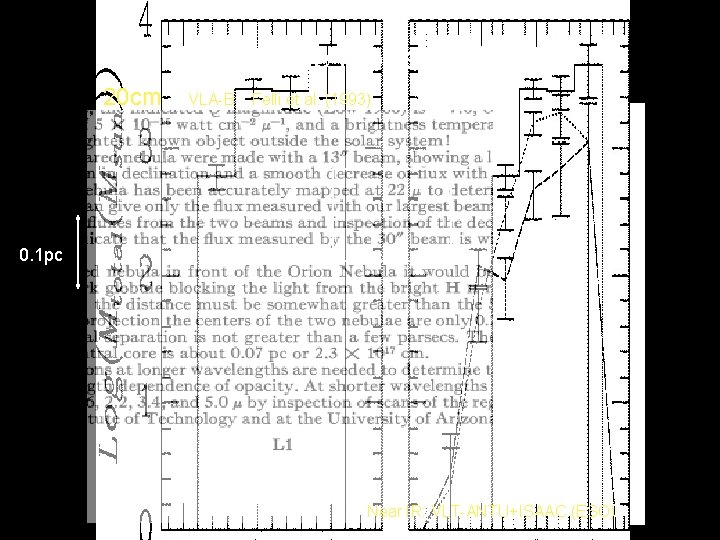
Testing the model in Orion 20 cm VLA-B Felli et al. (1993) 0. 1 pc Optical Near IR: VLT-ANTU+ISAAC (ESO)
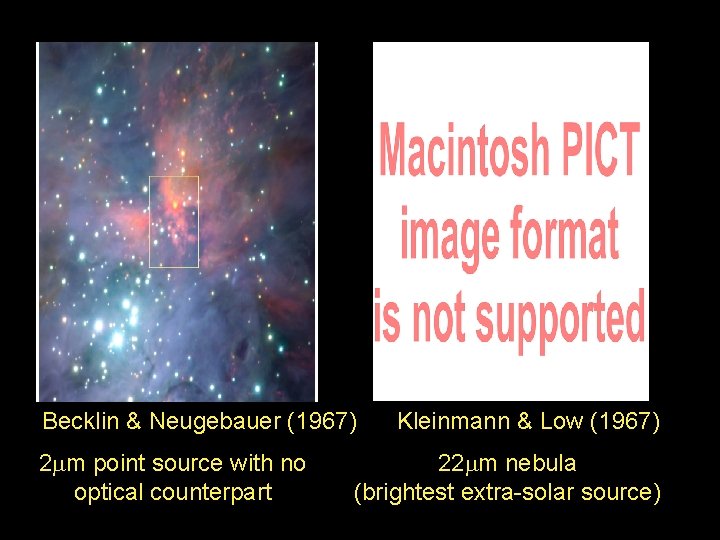
Becklin & Neugebauer (1967) 2 m point source with no optical counterpart Kleinmann & Low (1967) 22 m nebula (brightest extra-solar source)
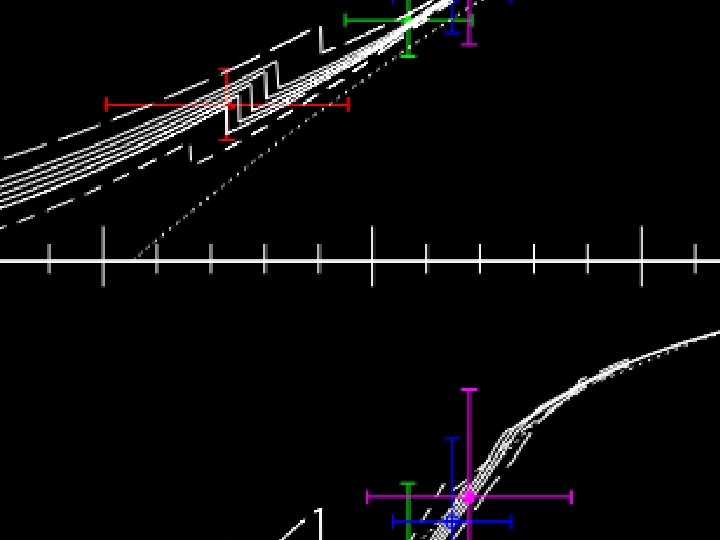
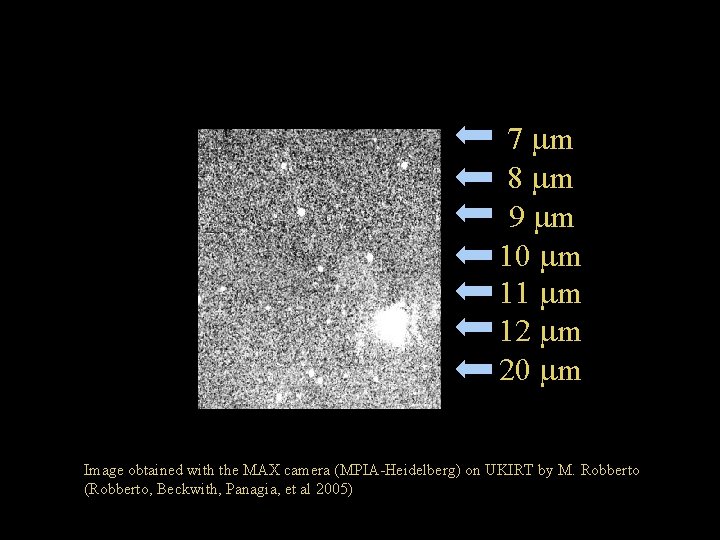
7 m 8 m 9 m 10 m 11 m 12 m 20 m Image obtained with the MAX camera (MPIA-Heidelberg) on UKIRT by M. Robberto (Robberto, Beckwith, Panagia, et al 2005)
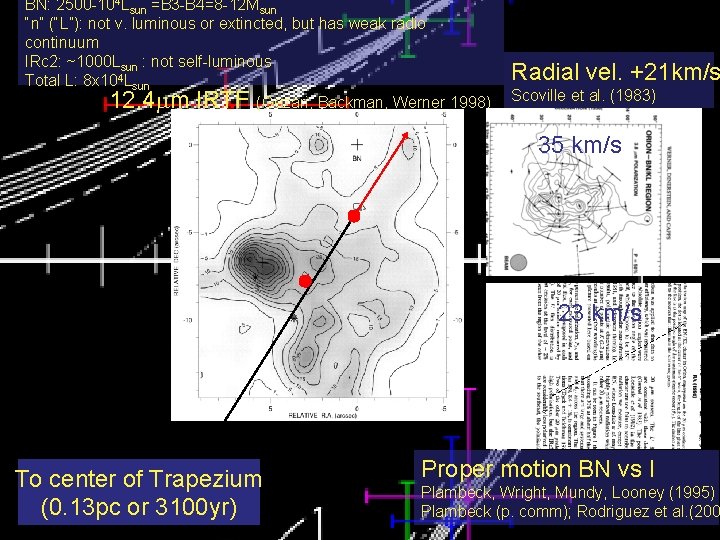
BN: 2500 -104 Lsun =B 3 -B 4=8 -12 Msun “n” (“L”): not v. luminous or extincted, but has weak radio continuum IRc 2: ~1000 Lsun : not self-luminous Total L: 8 x 104 Lsun 12. 4 m IRTF (Gezari, Backman, Werner 1998) Radial vel. +21 km/s Scoville et al. (1983) 35 km/s 23 km/s To center of Trapezium (0. 13 pc or 3100 yr) Proper motion BN vs I Plambeck, Wright, Mundy, Looney (1995) Plambeck (p. comm); Rodriguez et al. (200
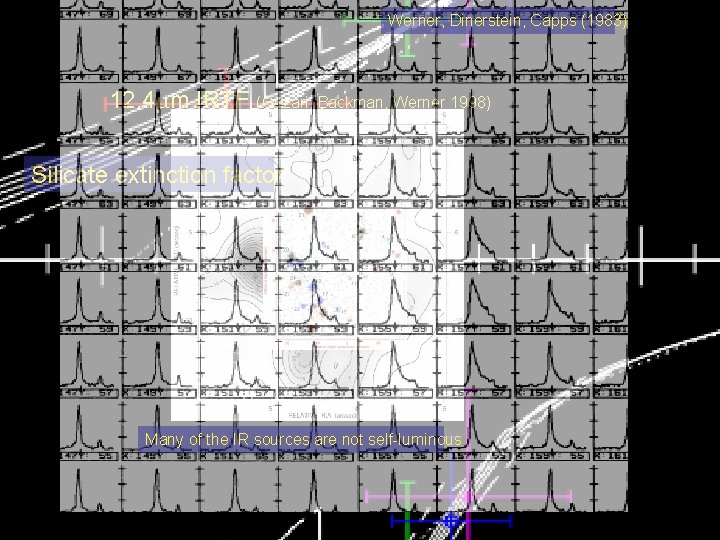
Werner, Dinerstein, Capps (1983) 12. 4 m IRTF (Gezari, Backman, Werner 1998) Silicate extinction factor Many of the IR sources are not self-luminous
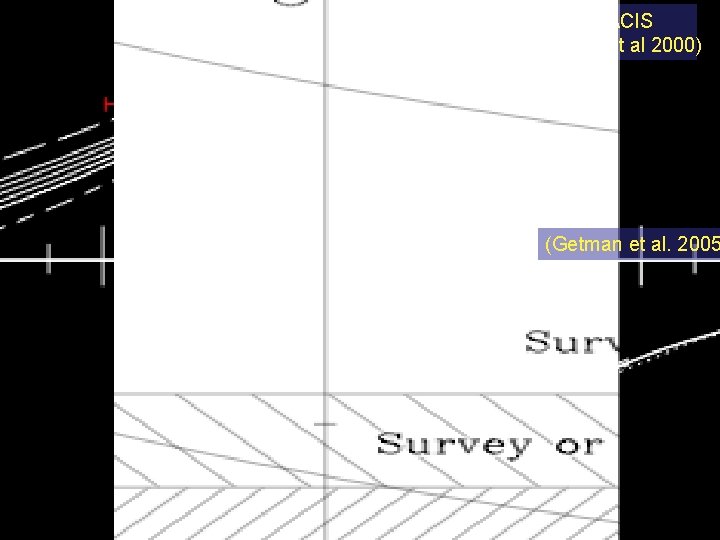
Chandra-ACIS (Garmire et al 2000) (Getman et al. 2005
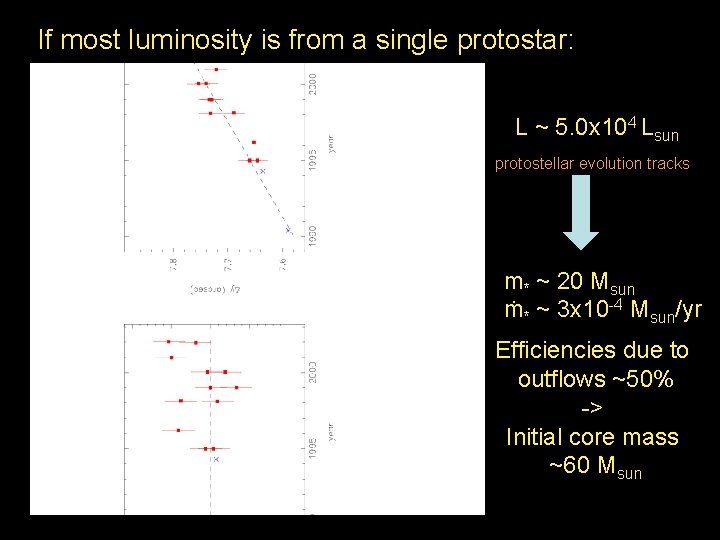
If most luminosity is from a single protostar: L ~ 5. 0 x 104 Lsun protostellar evolution tracks m* ~ 20 Msun. m* ~ 3 x 10 -4 Msun/yr Efficiencies due to outflows ~50% -> Initial core mass ~60 Msun
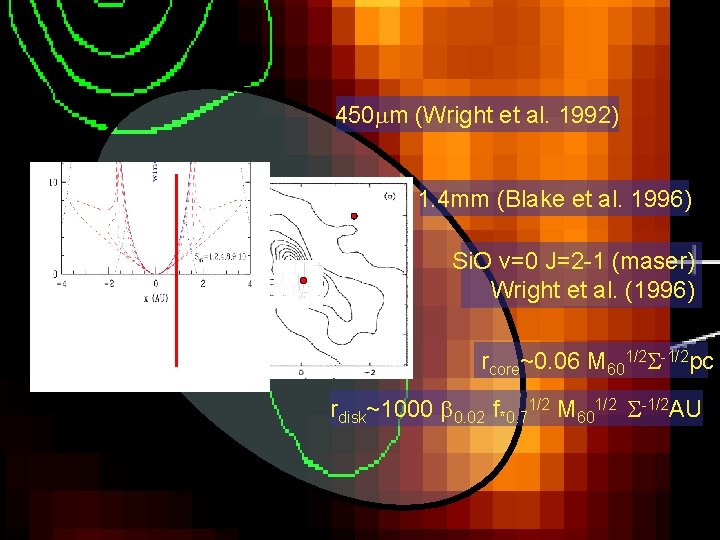
12. 4 m IRTF (Gezari, Backman, Werner 1998) 450 m (Wright et al. 1992) 1. 4 mm (Blake et al. 1996) Si. O v=0 J=2 -1 (maser) Wright et al. (1996) rcore~0. 06 M 601/2 -1/2 pc rdisk~1000 0. 02 f*0. 71/2 M 601/2 -1/2 AU
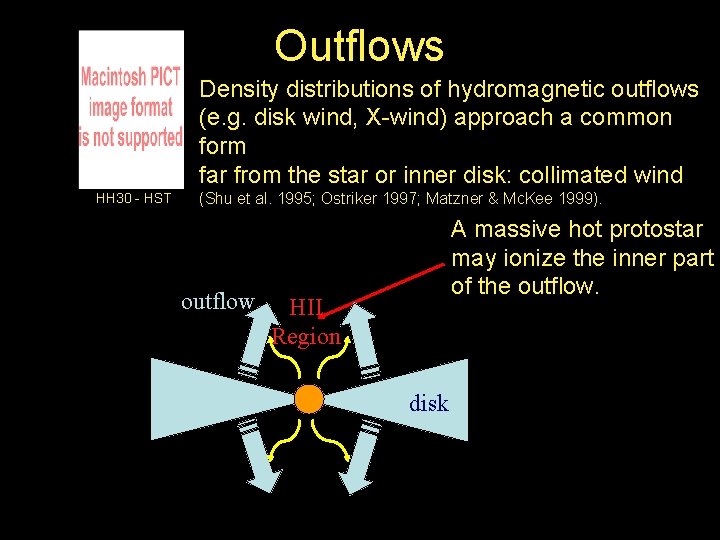
Outflows Density distributions of hydromagnetic outflows (e. g. disk wind, X-wind) approach a common form far from the star or inner disk: collimated wind HH 30 - HST (Shu et al. 1995; Ostriker 1997; Matzner & Mc. Kee 1999). outflow A massive hot protostar may ionize the inner part of the outflow. HII Region disk
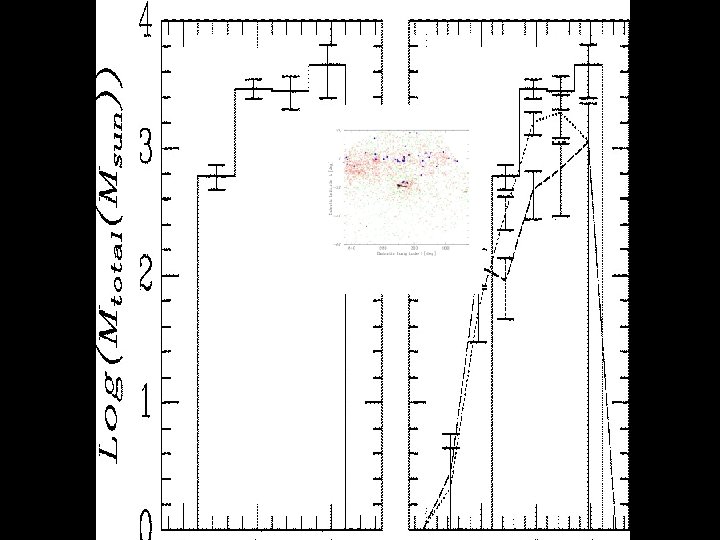
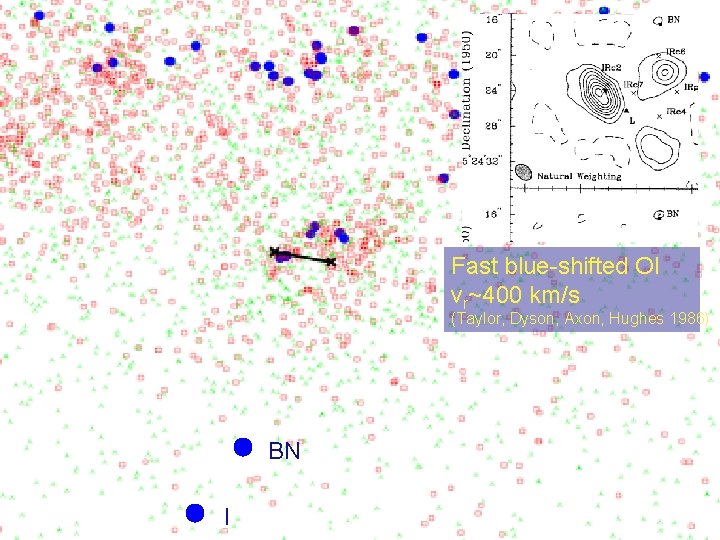
Fast blue-shifted OI vr~400 km/s (Taylor, Dyson, Axon, Hughes 1986) BN I
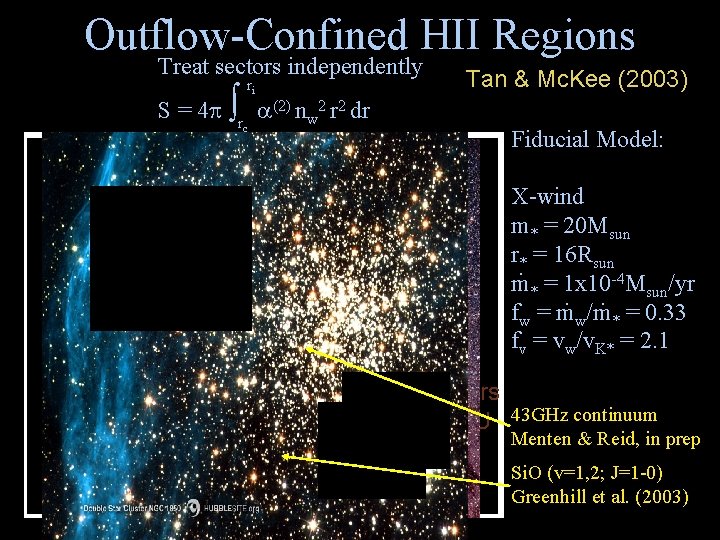
Outflow-Confined HII Regions Treat sectors independently Radio Spectrum: thermal bremsstraulung S = 4 ∫ ri rc (2) nw 2 r 2 dr Tan & Mc. Kee (2003) Fiducial Model: X-wind m* = 20 Msun r* = 16 Rsun. m* = 1 x 10 -4 Msun/yr. . fw = mw/m* = 0. 33 fv = vw/v. K* = 2. 1 Radio source “I” appears elongated (0. 145”=65 AU 43 GHz continuum Menten & Reid, in prep by <0. 085”) at 22 GHz (K. Menten, priv. comm) Si. O (v=1, 2; J=1 -0) Greenhill et al. (2003)
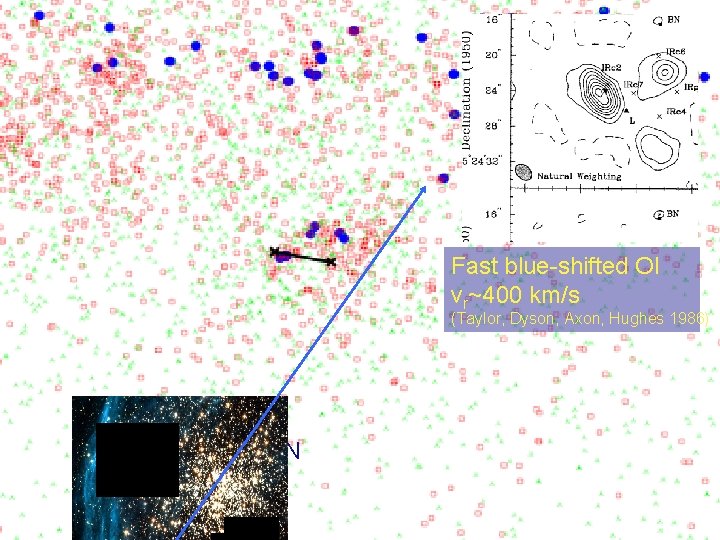
Fast blue-shifted OI vr~400 km/s (Taylor, Dyson, Axon, Hughes 1986) BN I
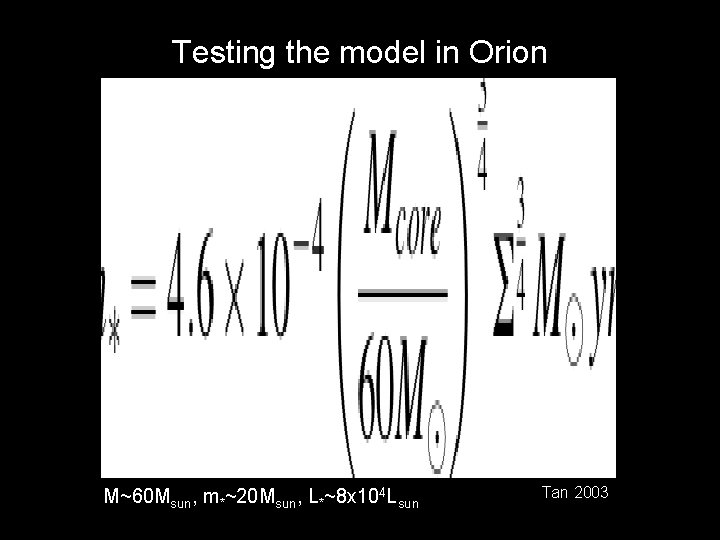
Testing the model in Orion Near IR (VLT: ANTU+ISAAC) M~60 Msun, m*~20 Msun, L*~8 x 104 Lsun Tan 2003
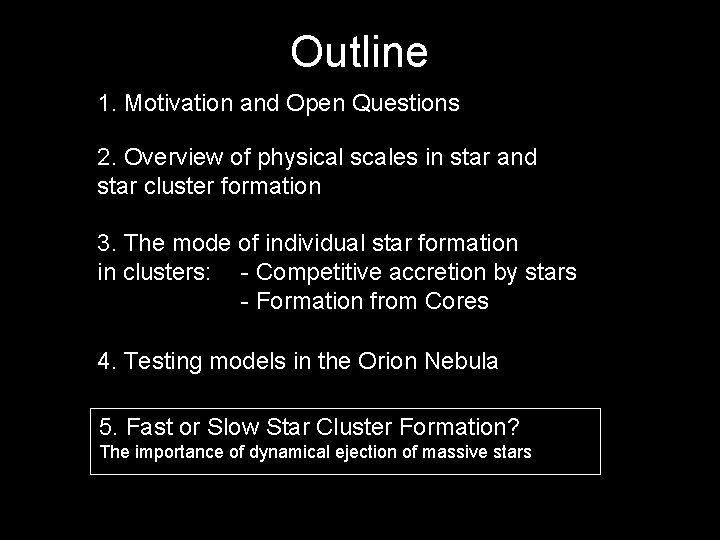
Outline 1. Motivation and Open Questions 2. Overview of physical scales in star and star cluster formation 3. The mode of individual star formation in clusters: - Competitive accretion by stars - Formation from Cores 4. Testing models in the Orion Nebula 5. Fast or Slow Star Cluster Formation? The importance of dynamical ejection of massive stars
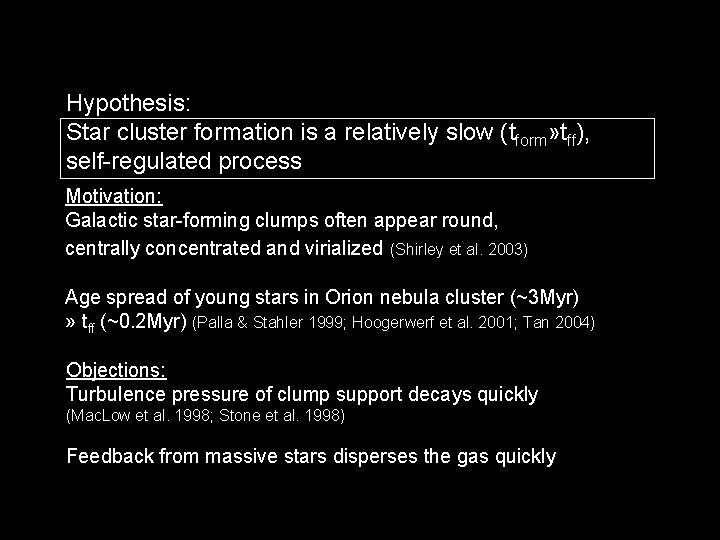
Hypothesis: Star cluster formation is a relatively slow (tform» tff), self-regulated process Motivation: Galactic star-forming clumps often appear round, centrally concentrated and virialized (Shirley et al. 2003) Age spread of young stars in Orion nebula cluster (~3 Myr) » tff (~0. 2 Myr) (Palla & Stahler 1999; Hoogerwerf et al. 2001; Tan 2004) Objections: Turbulence pressure of clump support decays quickly (Mac. Low et al. 1998; Stone et al. 1998) Feedback from massive stars disperses the gas quickly
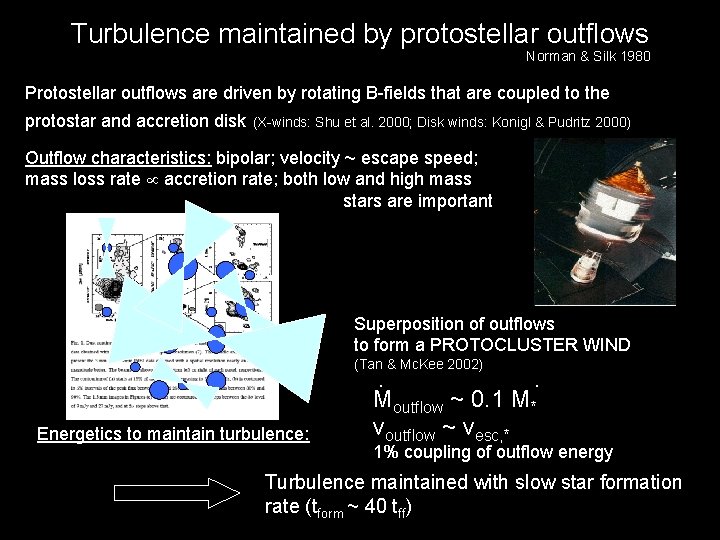
Turbulence maintained by protostellar outflows Norman & Silk 1980 Protostellar outflows are driven by rotating B-fields that are coupled to the protostar and accretion disk (X-winds: Shu et al. 2000; Disk winds: Konigl & Pudritz 2000) Outflow characteristics: bipolar; velocity ~ escape speed; mass loss rate accretion rate; both low and high mass stars are important Superposition of outflows to form a PROTOCLUSTER WIND (Tan & Mc. Kee 2002) Energetics to maintain turbulence: . . Moutflow ~ 0. 1 M* voutflow ~ vesc, * 1% coupling of outflow energy Turbulence maintained with slow star formation rate (tform ~ 40 tff)
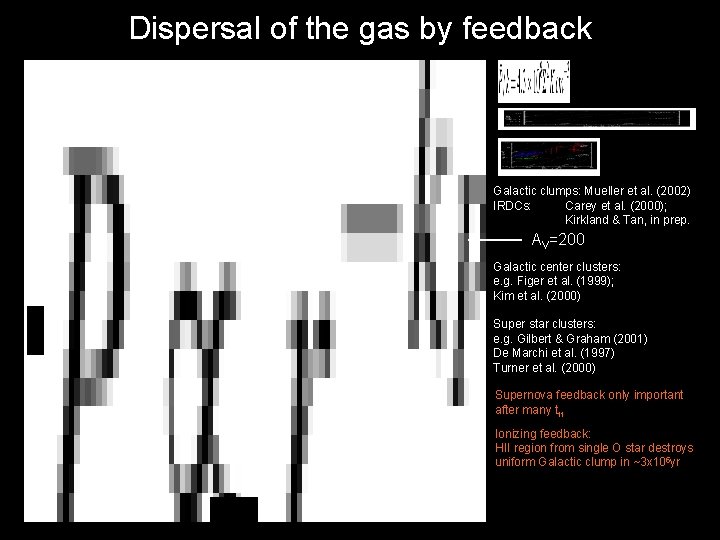
Dispersal of the gas by feedback Galactic clumps: Mueller et al. (2002) IRDCs: Carey et al. (2000); Kirkland & Tan, in prep. AV=200 Galactic center clusters: e. g. Figer et al. (1999); Kim et al. (2000) Super star clusters: e. g. Gilbert & Graham (2001) De Marchi et al. (1997) Turner et al. (2000) Supernova feedback only important after many tff Ionizing feedback: HII region from single O star destroys uniform Galactic clump in ~3 x 105 yr
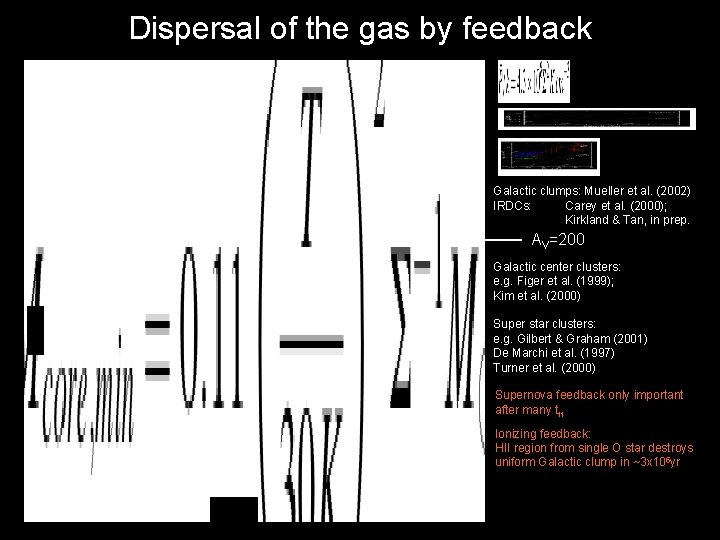
Dispersal of the gas by feedback Galactic clumps: Mueller et al. (2002) IRDCs: Carey et al. (2000); Kirkland & Tan, in prep. AV=200 Galactic center clusters: e. g. Figer et al. (1999); Kim et al. (2000) Super star clusters: e. g. Gilbert & Graham (2001) De Marchi et al. (1997) Turner et al. (2000) Supernova feedback only important after many tff Ionizing feedback: HII region from single O star destroys uniform Galactic clump in ~3 x 105 yr
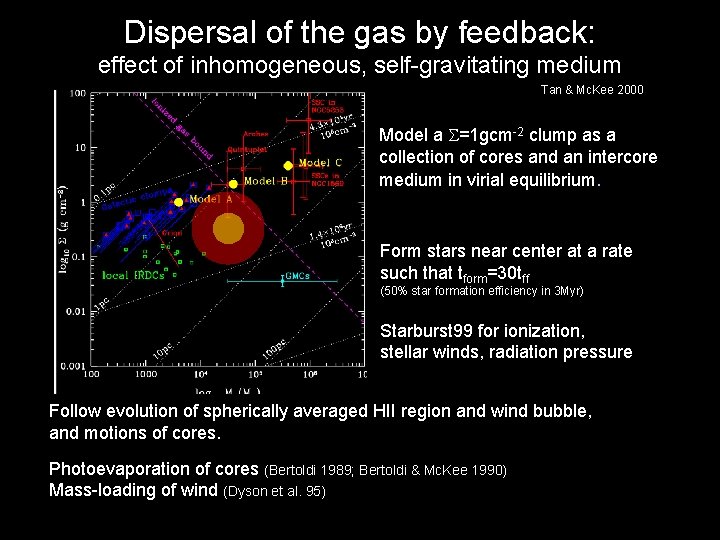
Dispersal of the gas by feedback: effect of inhomogeneous, self-gravitating medium Tan & Mc. Kee 2000 Model a =1 gcm-2 clump as a collection of cores and an intercore medium in virial equilibrium. Form stars near center at a rate such that tform=30 tff (50% star formation efficiency in 3 Myr) Starburst 99 for ionization, stellar winds, radiation pressure Follow evolution of spherically averaged HII region and wind bubble, and motions of cores. Photoevaporation of cores (Bertoldi 1989; Bertoldi & Mc. Kee 1990) Mass-loading of wind (Dyson et al. 95)
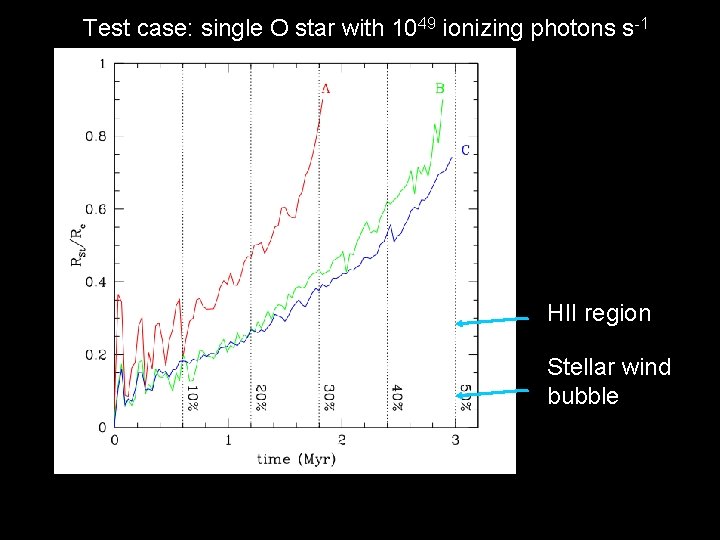
Test case: single O star with 1049 ionizing photons s-1 HII region Stellar wind bubble
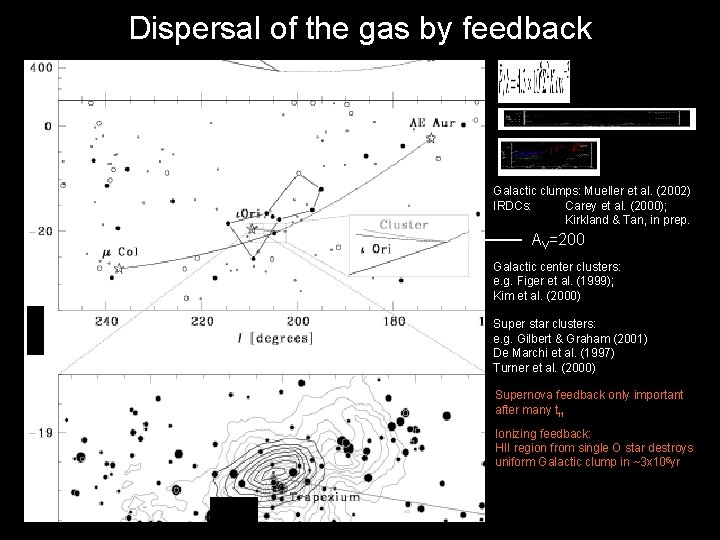
Dispersal of the gas by feedback Galactic clumps: Mueller et al. (2002) IRDCs: Carey et al. (2000); Kirkland & Tan, in prep. AV=200 Galactic center clusters: e. g. Figer et al. (1999); Kim et al. (2000) Super star clusters: e. g. Gilbert & Graham (2001) De Marchi et al. (1997) Turner et al. (2000) Supernova feedback only important after many tff Ionizing feedback: HII region from single O star destroys uniform Galactic clump in ~3 x 105 yr
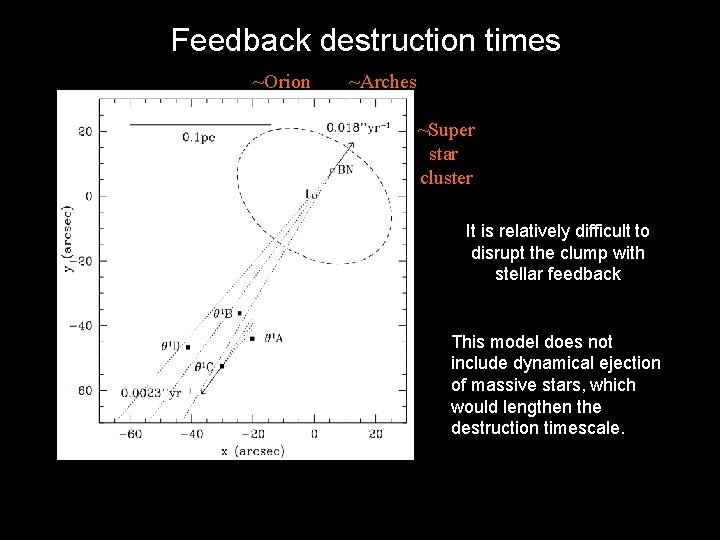
Feedback destruction times ~Orion ~Arches ~Super star cluster It is relatively difficult to disrupt the clump with stellar feedback This model does not include dynamical ejection of massive stars, which would lengthen the destruction timescale.
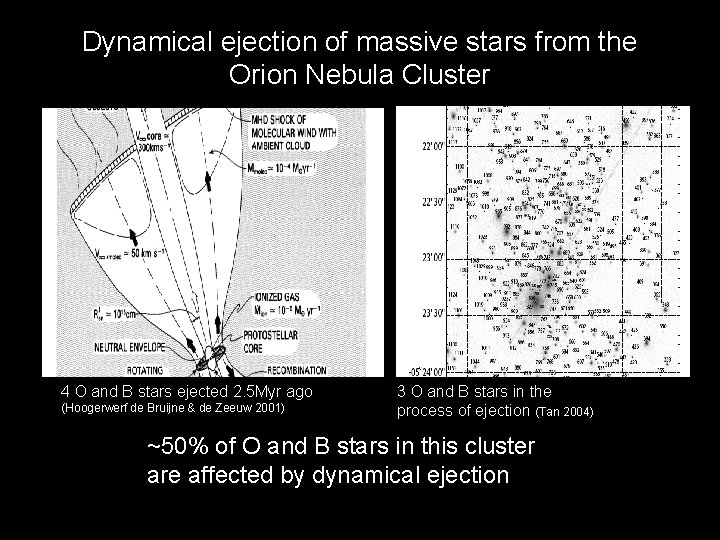
Dynamical ejection of massive stars from the Orion Nebula Cluster 4 O and B stars ejected 2. 5 Myr ago (Hoogerwerf de Bruijne & de Zeeuw 2001) 3 O and B stars in the process of ejection (Tan 2004) ~50% of O and B stars in this cluster are affected by dynamical ejection
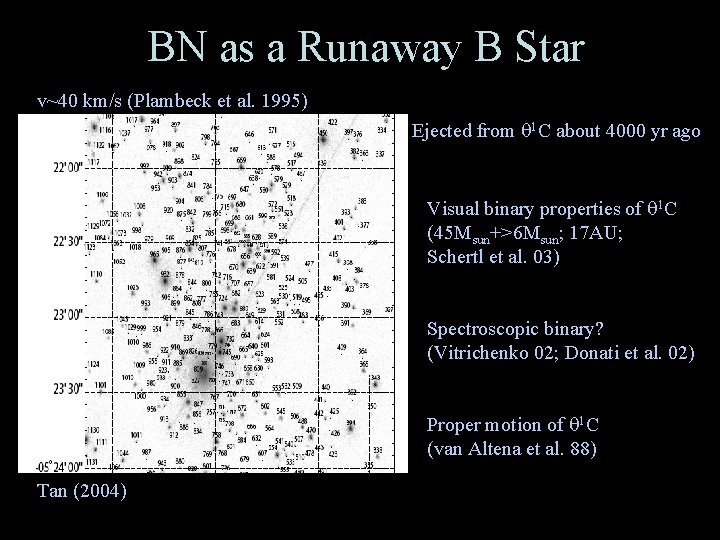
BN as a Runaway B Star v~40 km/s (Plambeck et al. 1995) Ejected from 1 C about 4000 yr ago Visual binary properties of 1 C (45 Msun+>6 Msun; 17 AU; Schertl et al. 03) Spectroscopic binary? (Vitrichenko 02; Donati et al. 02) Proper motion of 1 C (van Altena et al. 88) Tan (2004)
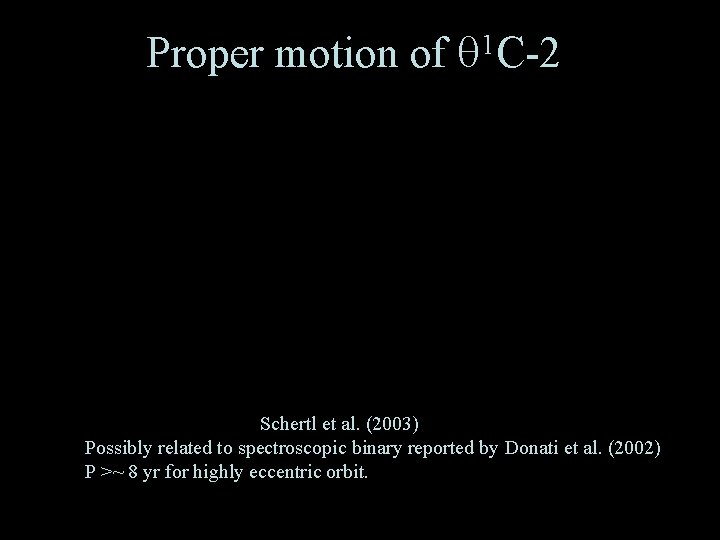
Proper motion of 1 C-2 Schertl et al. (2003) Possibly related to spectroscopic binary reported by Donati et al. (2002) P >~ 8 yr for highly eccentric orbit.
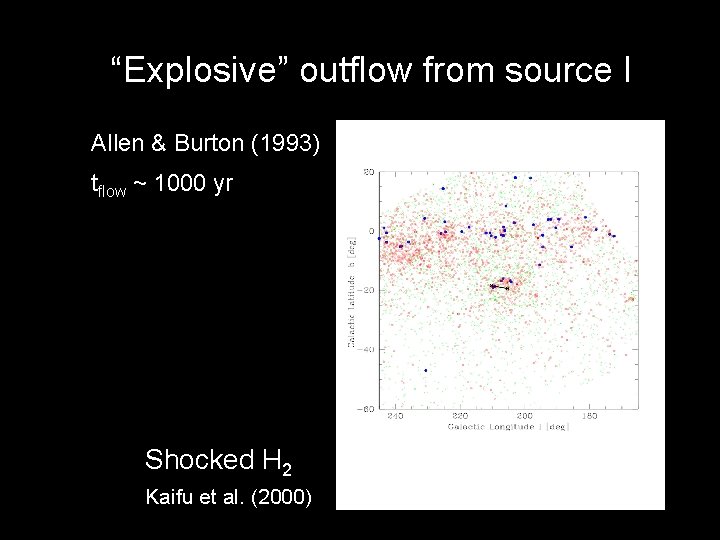
“Explosive” outflow from source I Allen & Burton (1993) tflow ~ 1000 yr Shocked H 2 Kaifu et al. (2000)
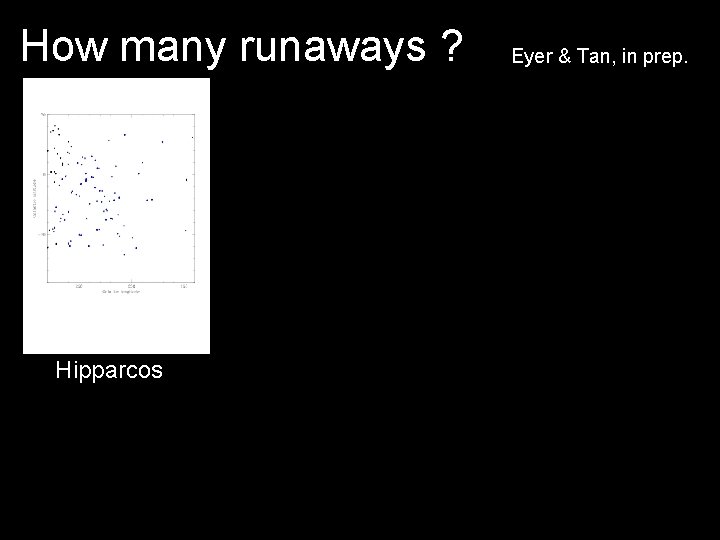
How many runaways ? Hipparcos Eyer & Tan, in prep.
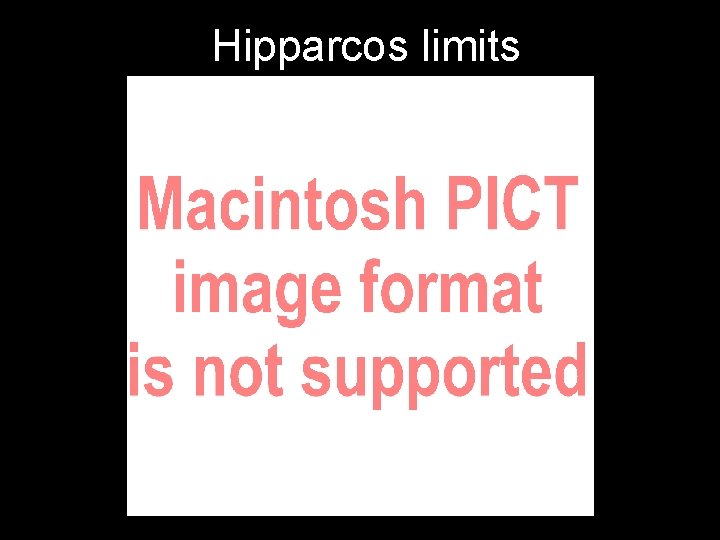
Hipparcos limits
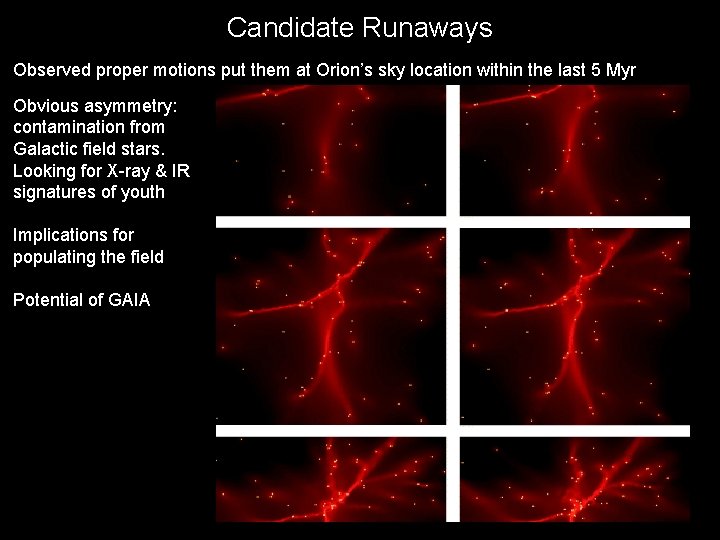
Candidate Runaways Observed proper motions put them at Orion’s sky location within the last 5 Myr Obvious asymmetry: contamination from Galactic field stars. Looking for X-ray & IR signatures of youth Implications for populating the field Potential of GAIA
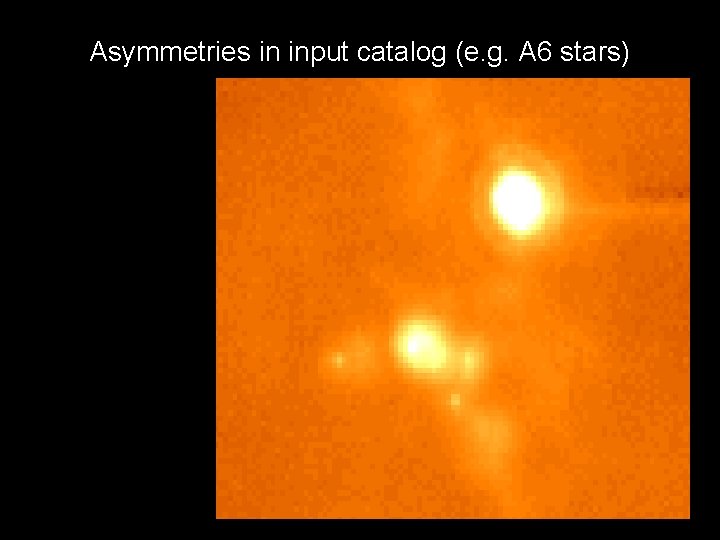
Asymmetries in input catalog (e. g. A 6 stars)
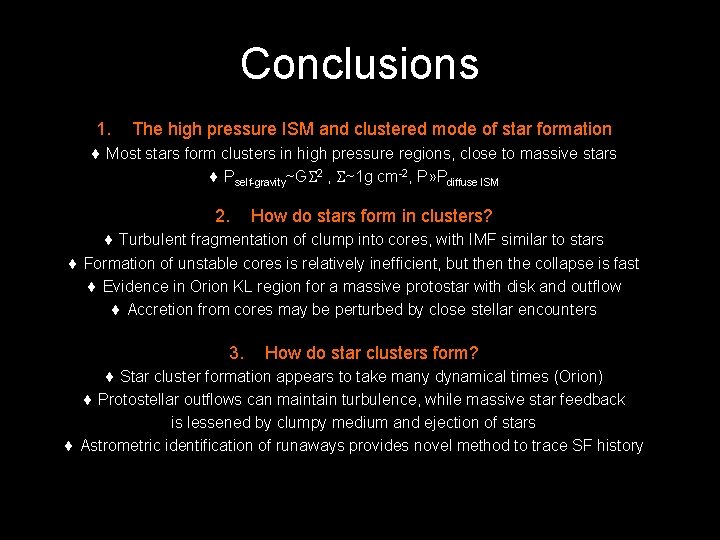
Conclusions 1. The high pressure ISM and clustered mode of star formation Most stars form clusters in high pressure regions, close to massive stars Pself-gravity~G 2 , ~1 g cm-2, P» Pdiffuse ISM 2. How do stars form in clusters? Turbulent fragmentation of clump into cores, with IMF similar to stars Formation of unstable cores is relatively inefficient, but then the collapse is fast Evidence in Orion KL region for a massive protostar with disk and outflow Accretion from cores may be perturbed by close stellar encounters 3. How do star clusters form? Star cluster formation appears to take many dynamical times (Orion) Protostellar outflows can maintain turbulence, while massive star feedback is lessened by clumpy medium and ejection of stars Astrometric identification of runaways provides novel method to trace SF history
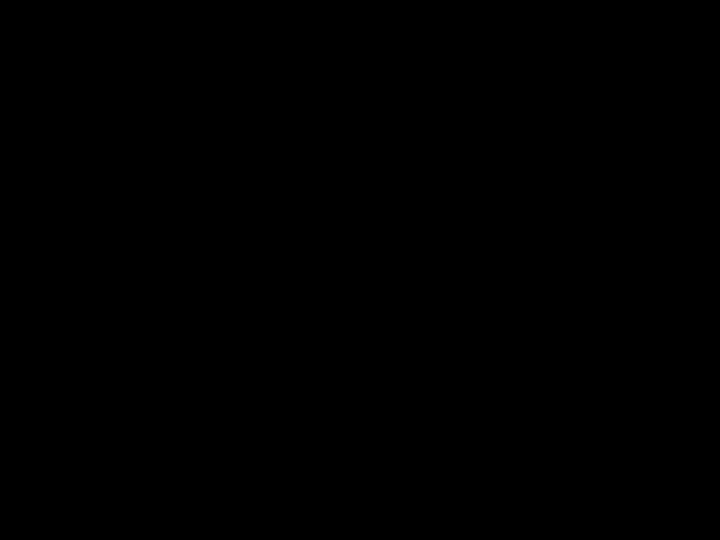
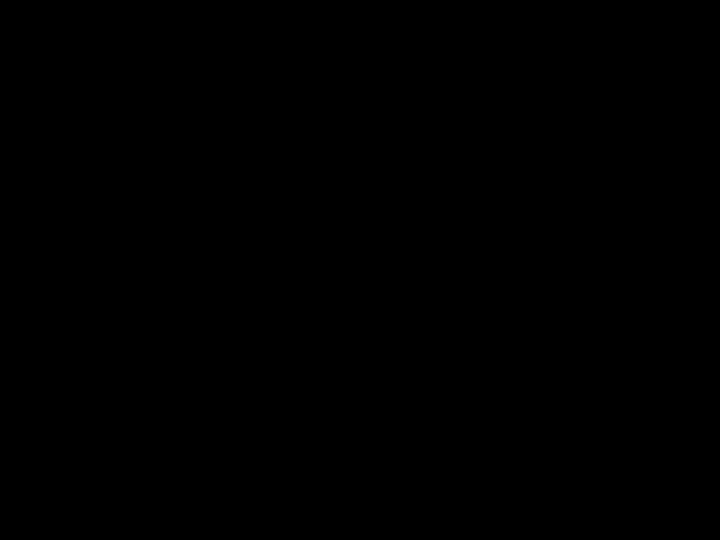
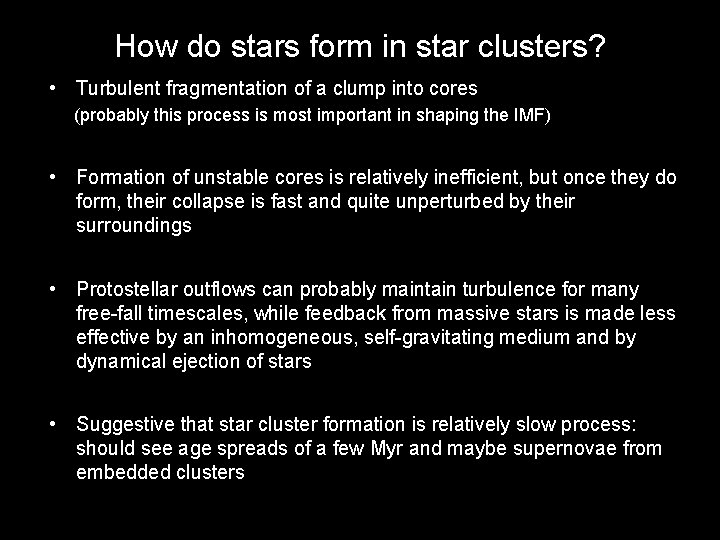
How do stars form in star clusters? • Turbulent fragmentation of a clump into cores (probably this process is most important in shaping the IMF) • Formation of unstable cores is relatively inefficient, but once they do form, their collapse is fast and quite unperturbed by their surroundings • Protostellar outflows can probably maintain turbulence for many free-fall timescales, while feedback from massive stars is made less effective by an inhomogeneous, self-gravitating medium and by dynamical ejection of stars • Suggestive that star cluster formation is relatively slow process: should see age spreads of a few Myr and maybe supernovae from embedded clusters
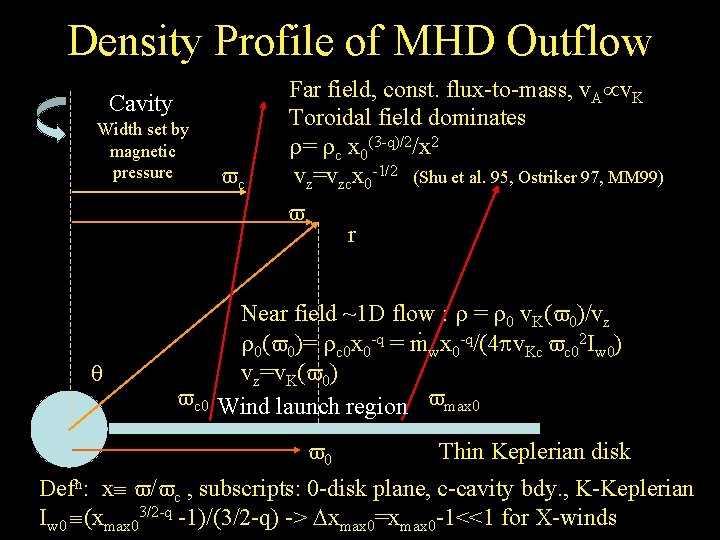
Density Profile of MHD Outflow Cavity Width set by magnetic pressure c Far field, const. flux-to-mass, v. A v. K Toroidal field dominates = c x 0(3 -q)/2/x 2 vz=vzcx 0 -1/2 (Shu et al. 95, Ostriker 97, MM 99) r Near field ~1 D flow : = 0 v. K( 0)/vz. -q 0( 0)= c 0 x 0 = mwx 0 -q/(4 v. Kc c 02 Iw 0) vz=v. K( 0) c 0 Wind launch region max 0 0 Thin Keplerian disk Defn: x / c , subscripts: 0 -disk plane, c-cavity bdy. , K-Keplerian Iw 0 (xmax 03/2 -q -1)/(3/2 -q) -> xmax 0=xmax 0 -1<<1 for X-winds
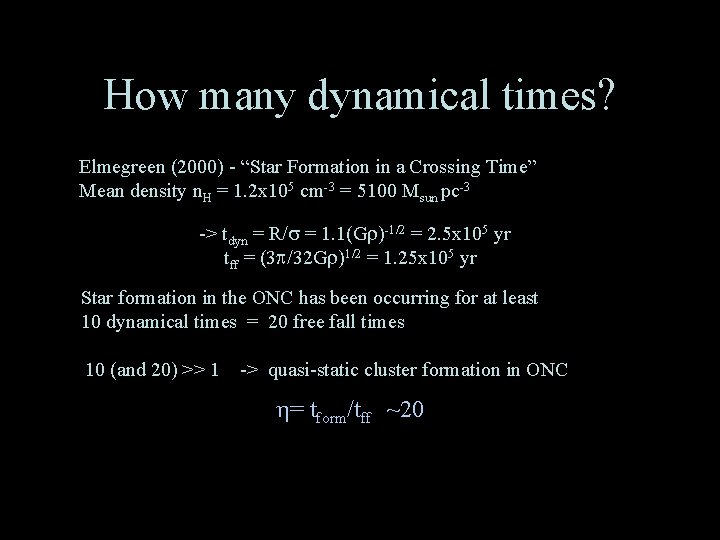
How many dynamical times? Elmegreen (2000) - “Star Formation in a Crossing Time” Mean density n. H = 1. 2 x 105 cm-3 = 5100 Msun pc-3 -> tdyn = R/ = 1. 1(G )-1/2 = 2. 5 x 105 yr tff = (3 /32 G )1/2 = 1. 25 x 105 yr Star formation in the ONC has been occurring for at least 10 dynamical times = 20 free fall times 10 (and 20) >> 1 -> quasi-static cluster formation in ONC = tform/tff ~20
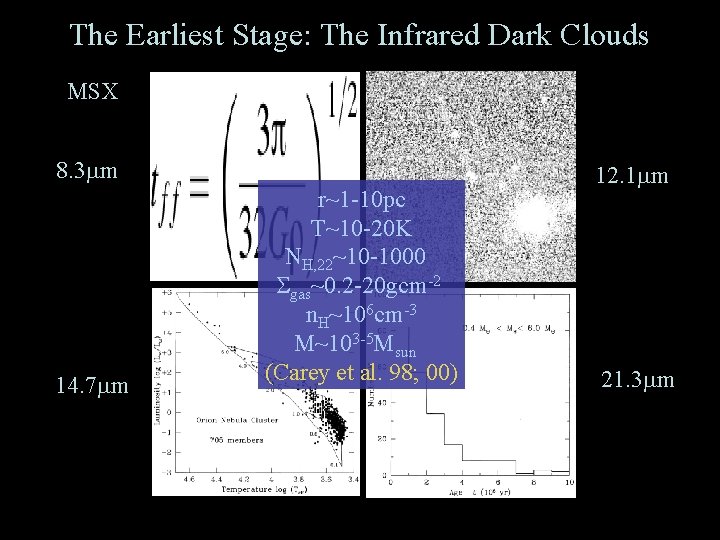
The Earliest Stage: The Infrared Dark Clouds MSX 8. 3 m 14. 7 m r~1 -10 pc T~10 -20 K NH, 22~10 -1000 gas~0. 2 -20 gcm-2 n. H~106 cm-3 M~103 -5 Msun (Carey et al. 98; 00) 12. 1 m 21. 3 m
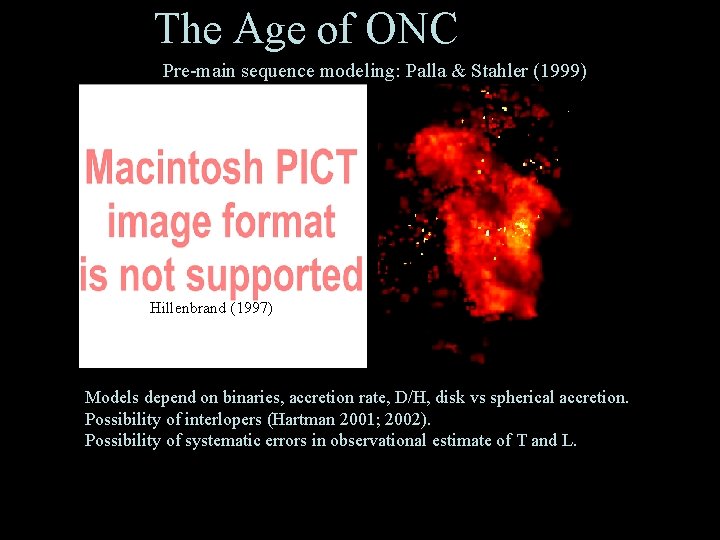
The Age of ONC Pre-main sequence modeling: Palla & Stahler (1999) Hillenbrand (1997) Models depend on binaries, accretion rate, D/H, disk vs spherical accretion. Possibility of interlopers (Hartman 2001; 2002). Possibility of systematic errors in observational estimate of T and L.
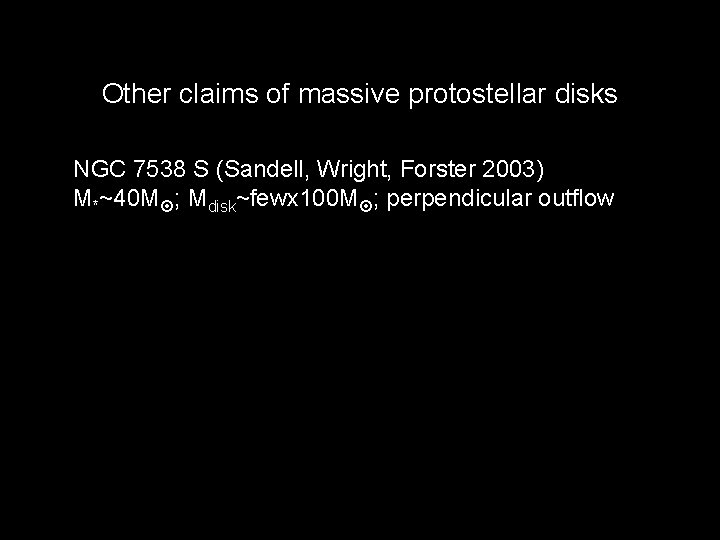
Other claims of massive protostellar disks NGC 7538 S (Sandell, Wright, Forster 2003) M*~40 M ; Mdisk~fewx 100 M ; perpendicular outflow
Life cycle of a small star
Myrrh is mine its bitter perfume
Schnauzer dogs there are three sizes
Ao* algorithm in artificial intelligence
What does star (for star events) stand for
Star formation
Star formation
Star formation
Star formation
Formation initiale vs formation continue
Excessive use of ornamentation and massive proportions
Massive comparative and superlative
Thereoncewasawoman
Laccolith shape
Seabed massive sulphides (sms)
Massive transformative purpose
Massive transfusion protocol
Nellie hangs motionless by one hand from a clothesline
Cs 246 stanford
What are the complications of blood transfusion
Blood transfusion allergic reaction
Bambonite
Mining of massive datasets stanford
Massive hemothorax
Tension pneumothorax triad
Giant wood chipper
Hémiplégie massive proportionnelle
A small sports car collides head on with a massive truck
Mining of massive datasets solution
Massive transfusion protocol template
Stanford mining massive datasets
Massive io
Massive splenomegaly
Massive compact halo objects
Define emesis
Massive transfusion protocol template
Calcium infusion protocol
Why did stalin want to create a “buffer zone”?
Internetmultimedia org live stream
Endophytes are primary producers that live
Hát kết hợp bộ gõ cơ thể
Frameset trong html5
Bổ thể
Tỉ lệ cơ thể trẻ em
Voi kéo gỗ như thế nào
Chụp tư thế worms-breton
Bài hát chúa yêu trần thế alleluia
Các môn thể thao bắt đầu bằng từ đua
Thế nào là hệ số cao nhất
Các châu lục và đại dương trên thế giới
Cong thức tính động năng
Trời xanh đây là của chúng ta thể thơ
Mật thư tọa độ 5x5
101012 bằng
độ dài liên kết
Các châu lục và đại dương trên thế giới
Thể thơ truyền thống
Quá trình desamine hóa có thể tạo ra
Một số thể thơ truyền thống
Bàn tay mà dây bẩn
Vẽ hình chiếu vuông góc của vật thể sau
Nguyên nhân của sự mỏi cơ sinh 8
đặc điểm cơ thể của người tối cổ
V cc cc
Vẽ hình chiếu đứng bằng cạnh của vật thể
Vẽ hình chiếu vuông góc của vật thể sau
Thẻ vin