The Cellular Concept Cellular radio systems accommodate a
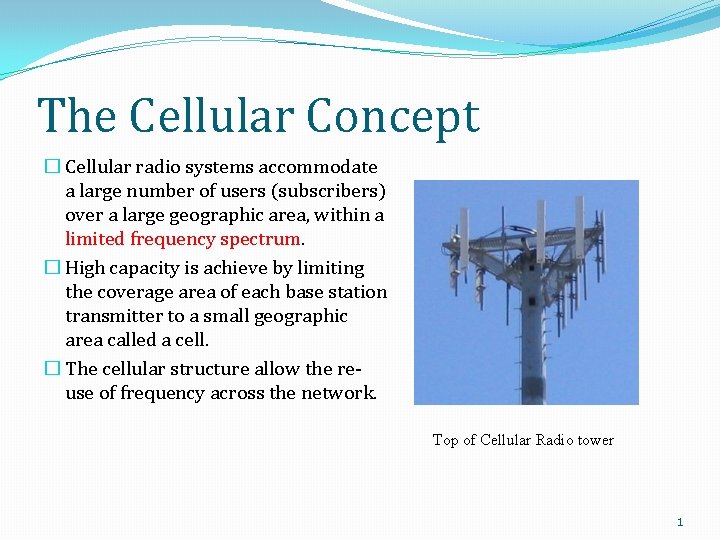
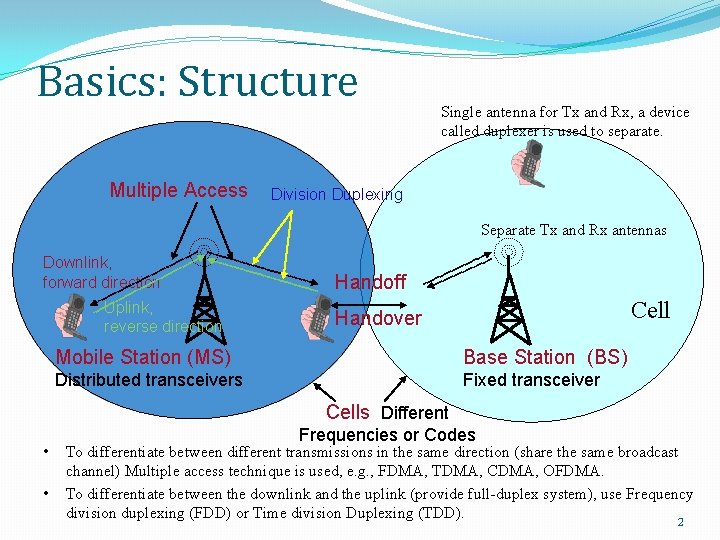
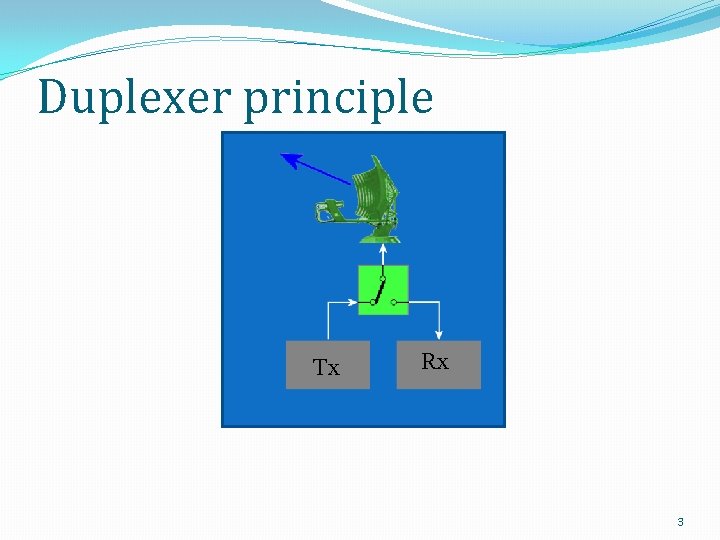
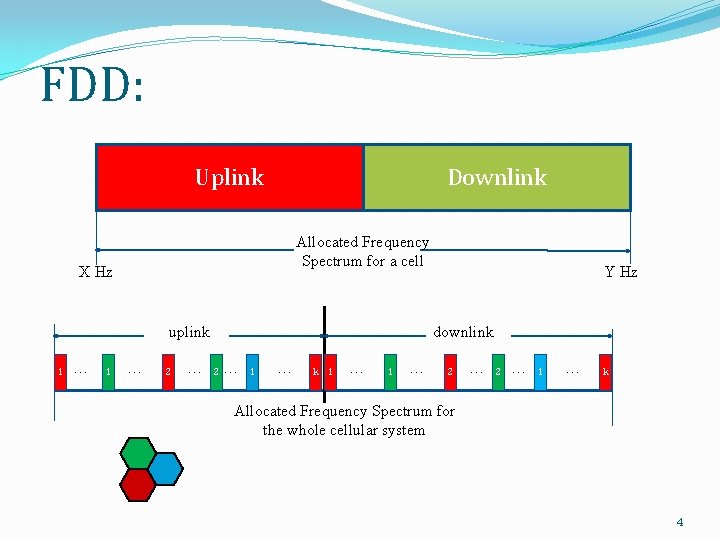
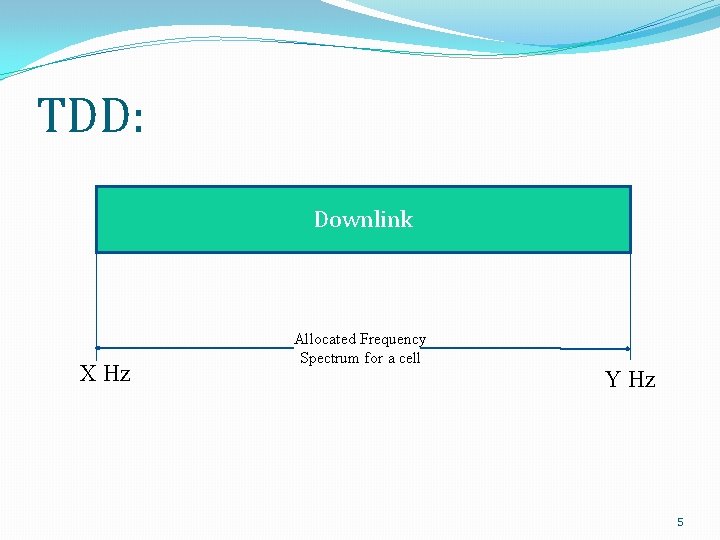
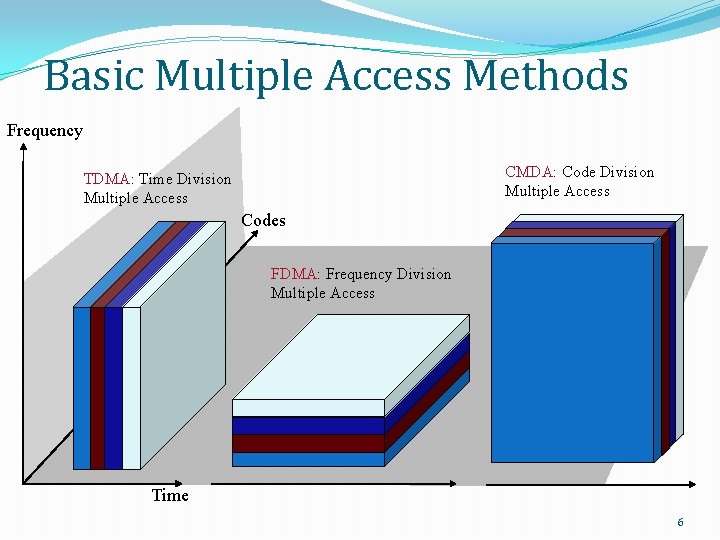
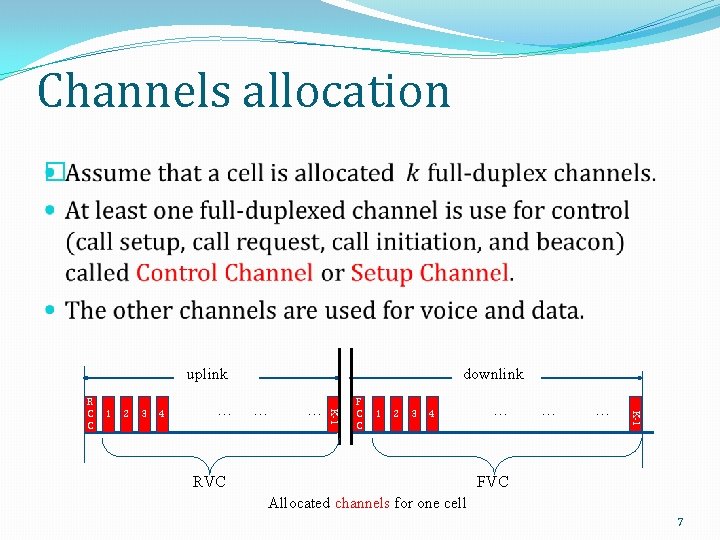
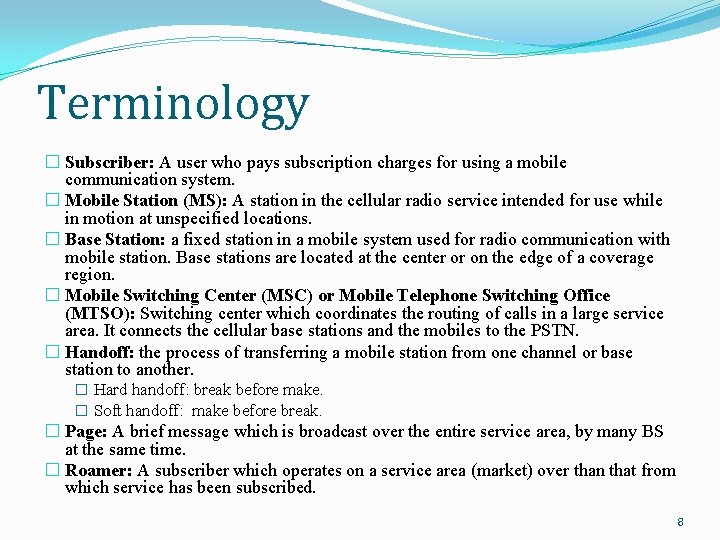
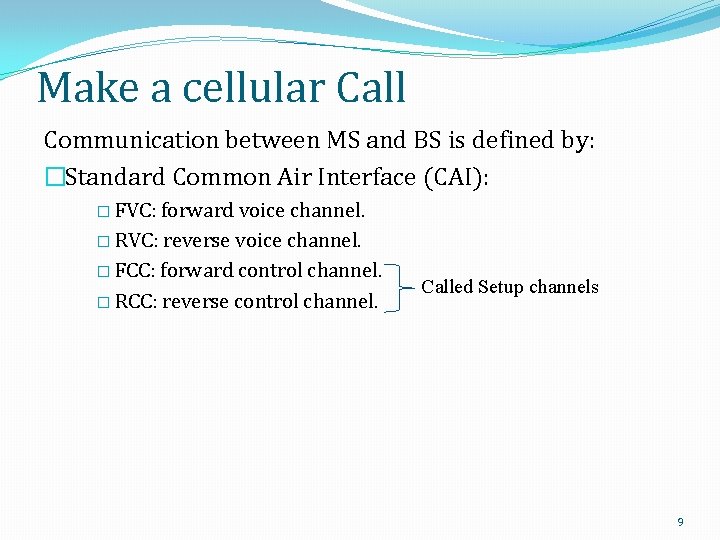
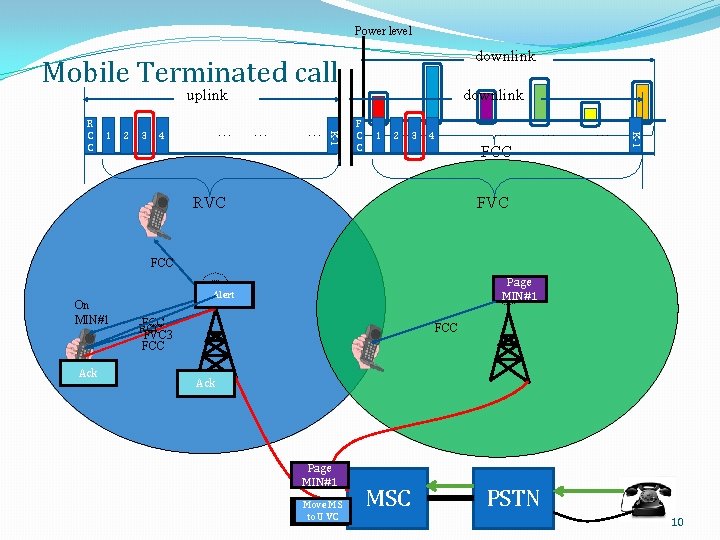
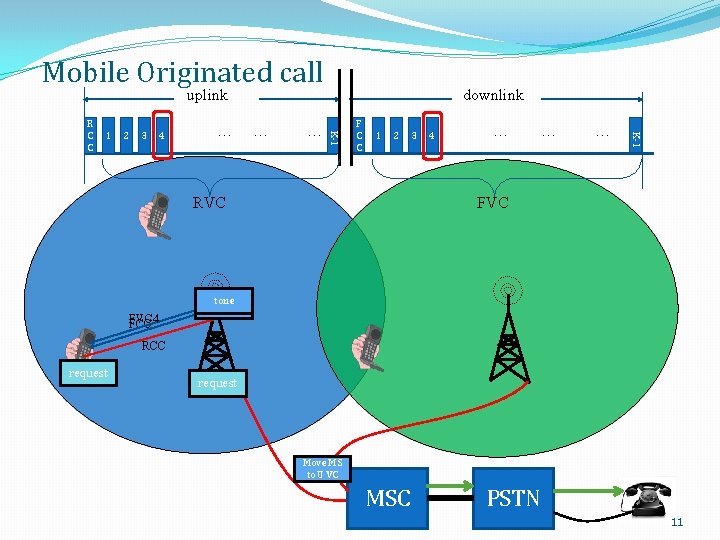
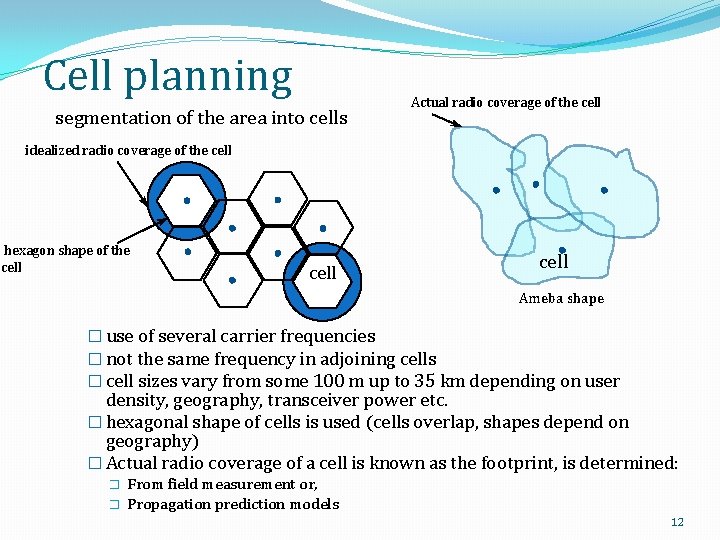
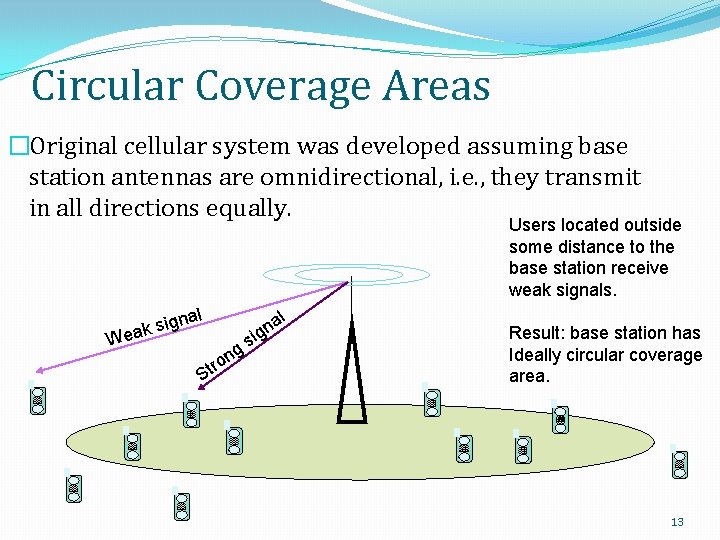
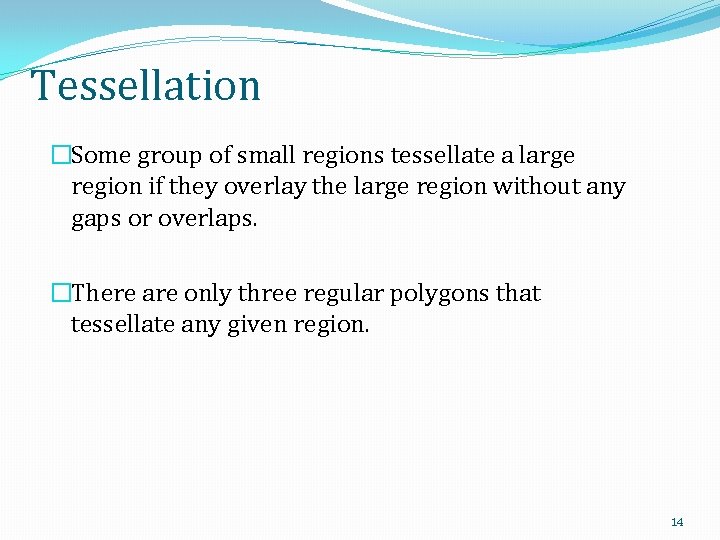
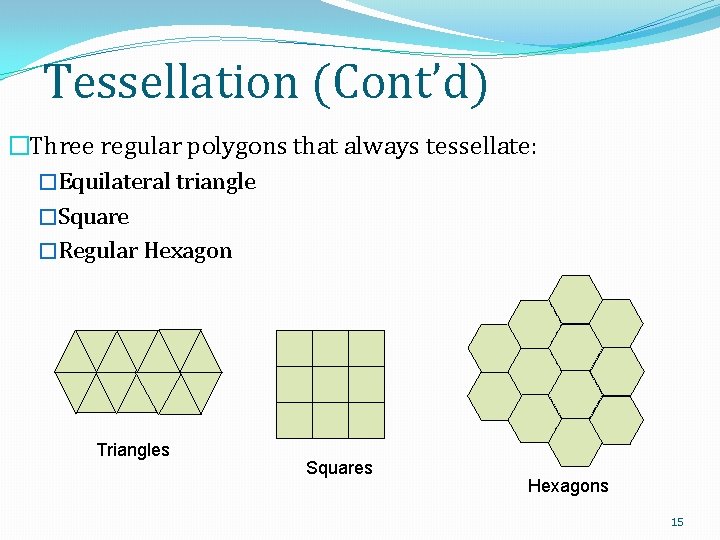
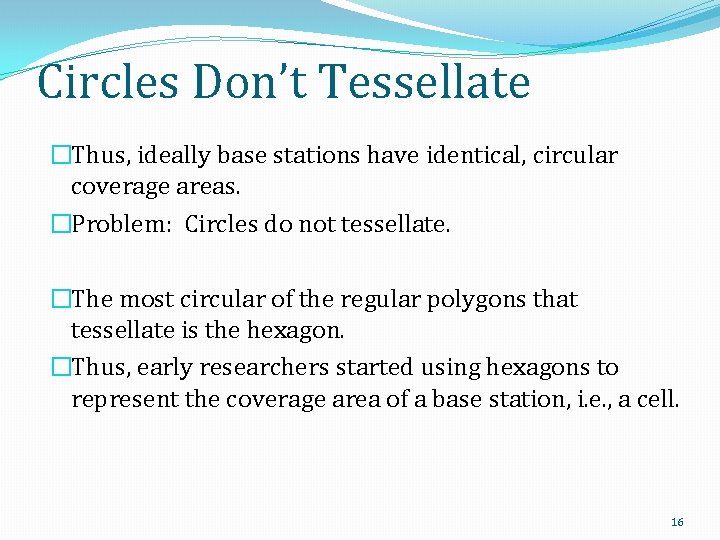
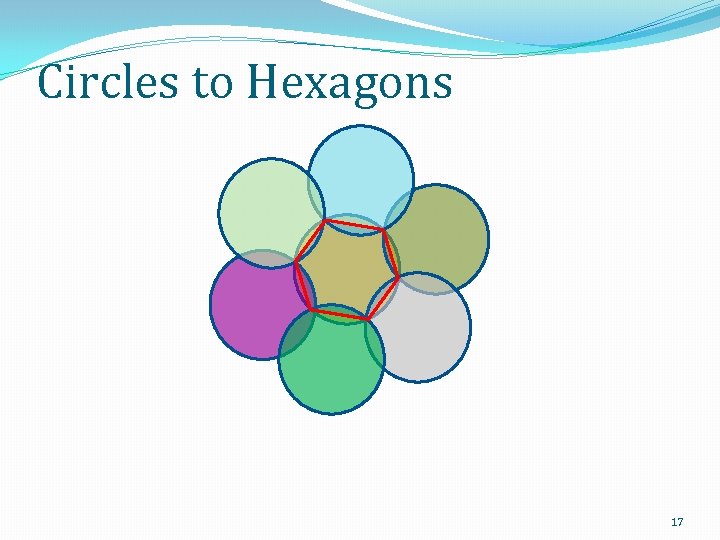
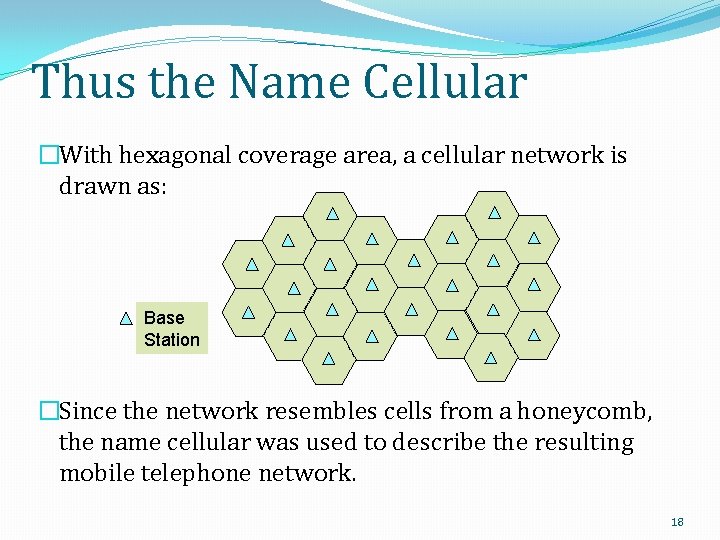
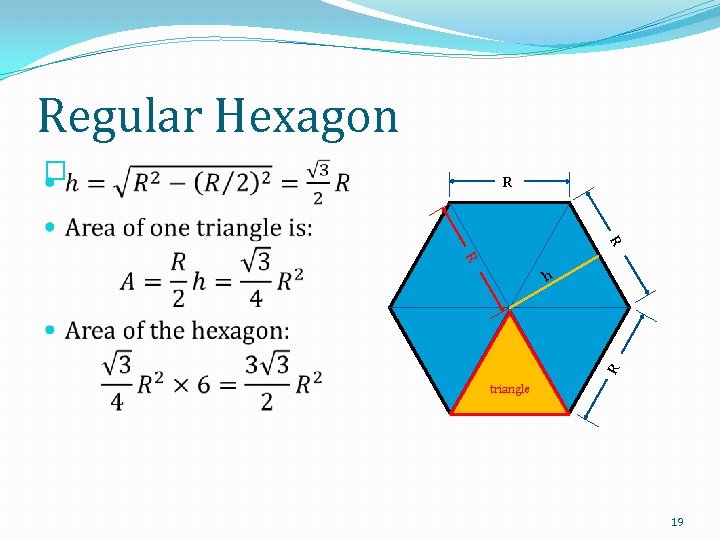
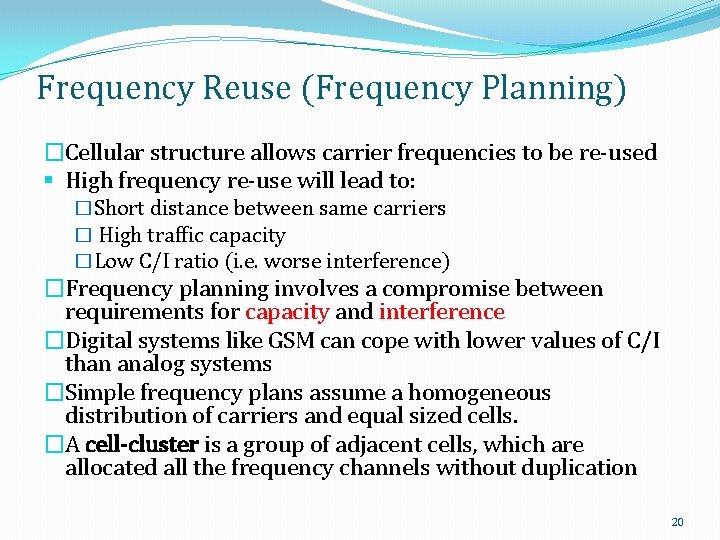
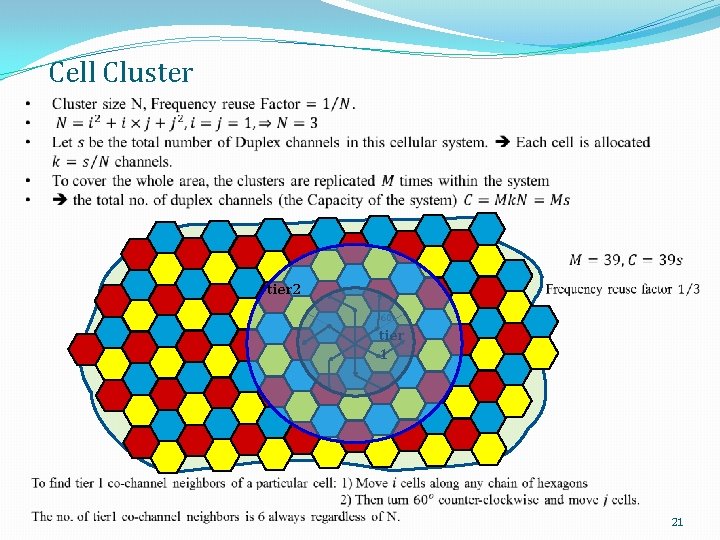
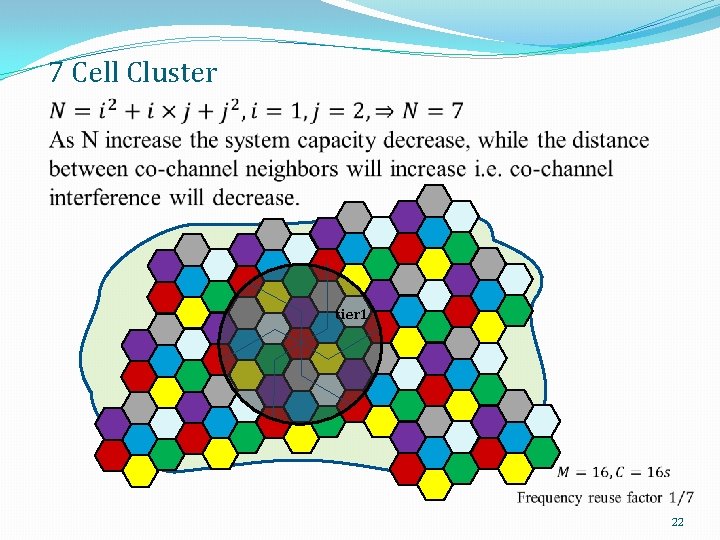
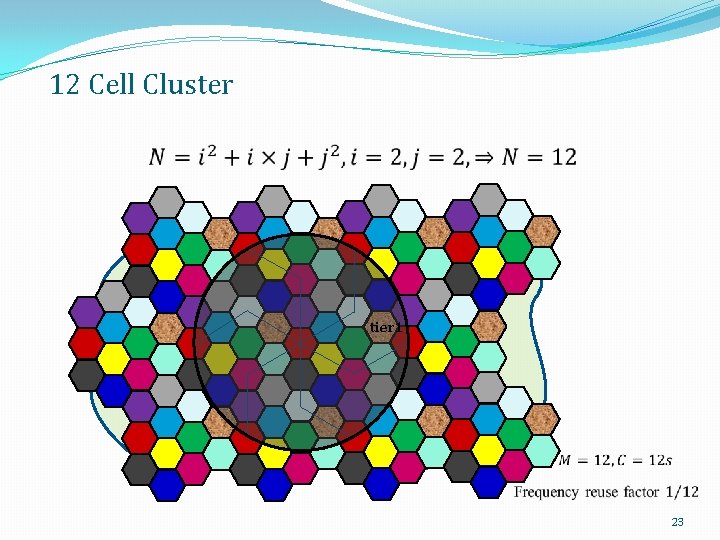
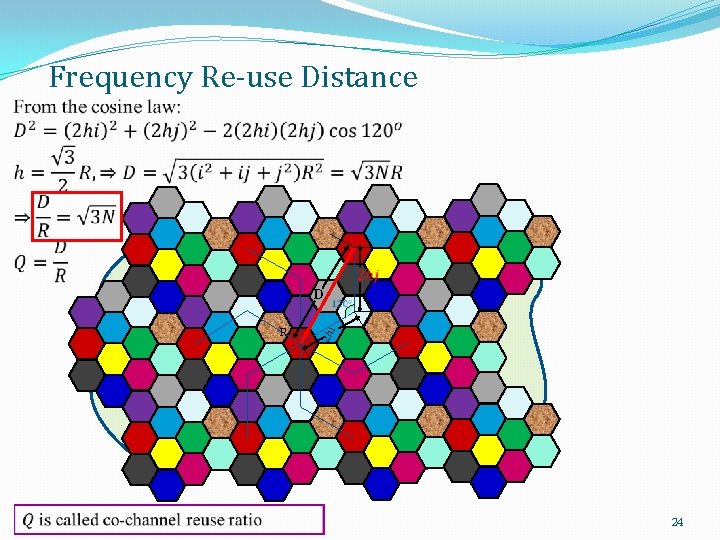
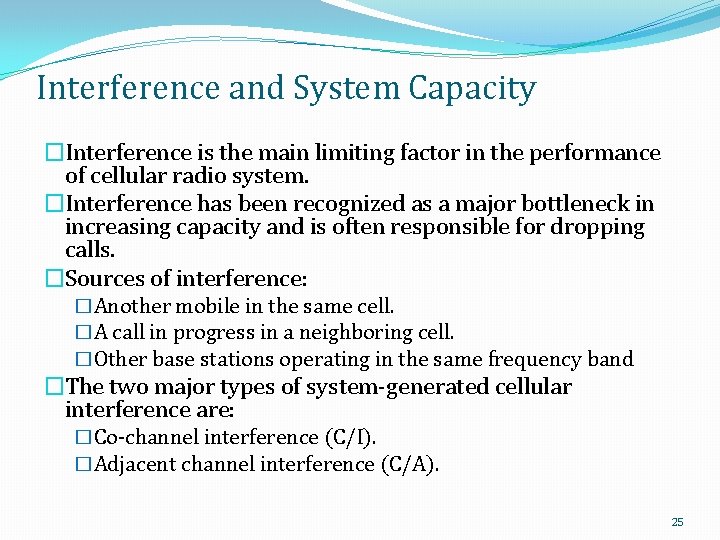
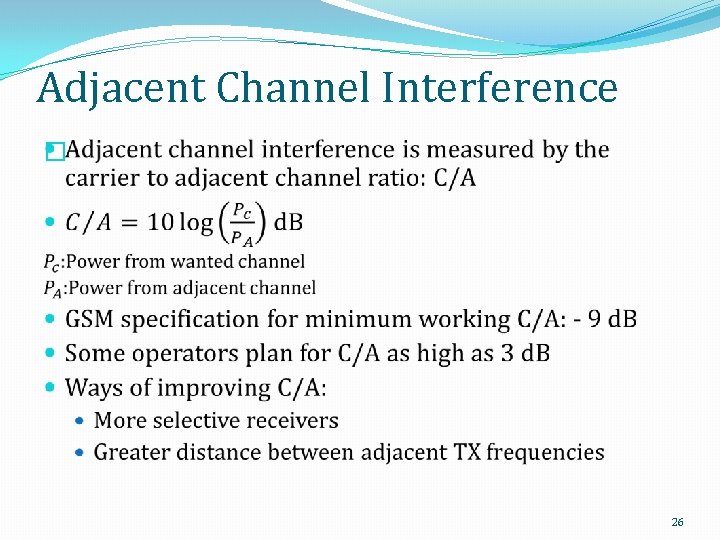
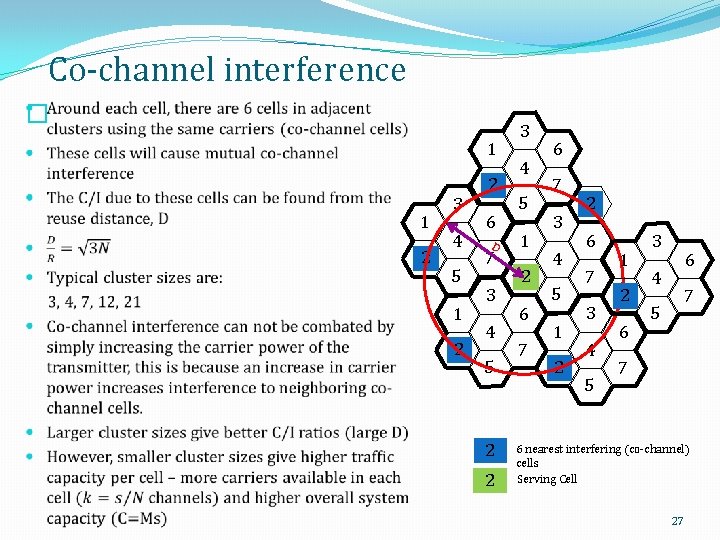
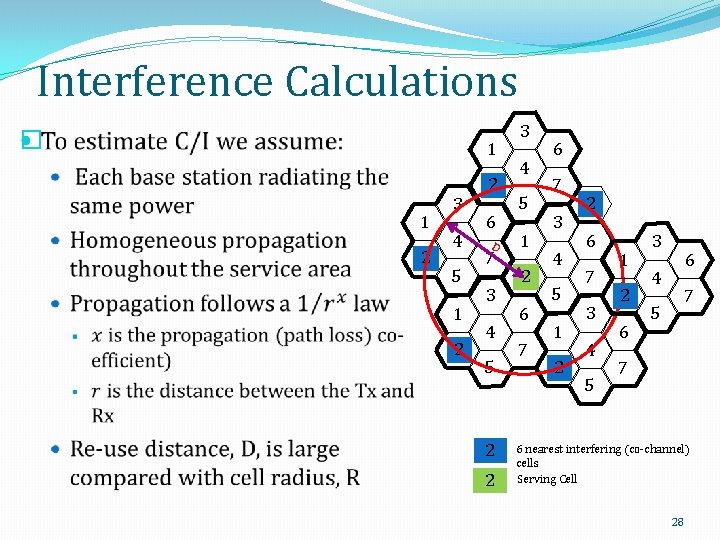
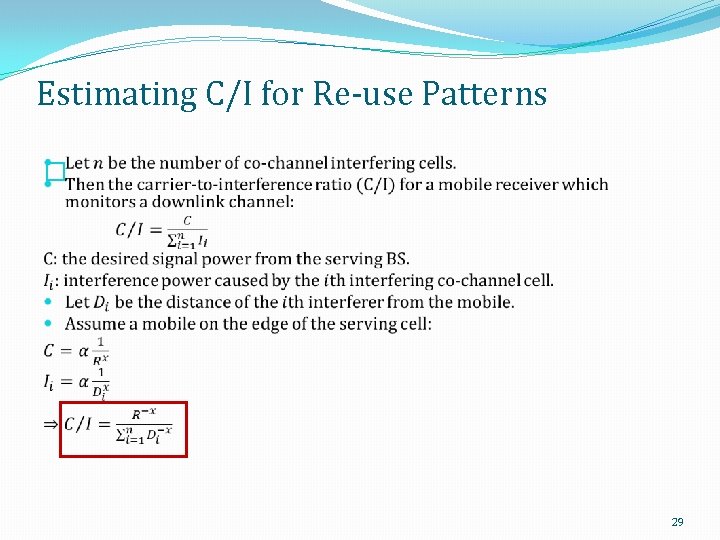
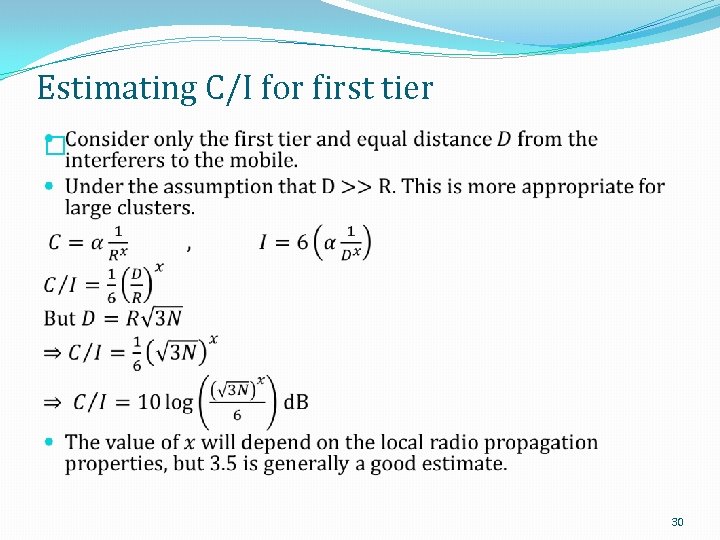
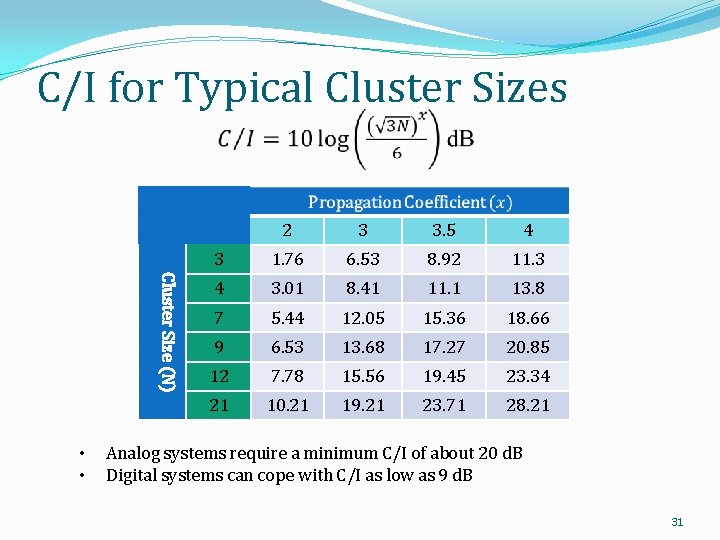
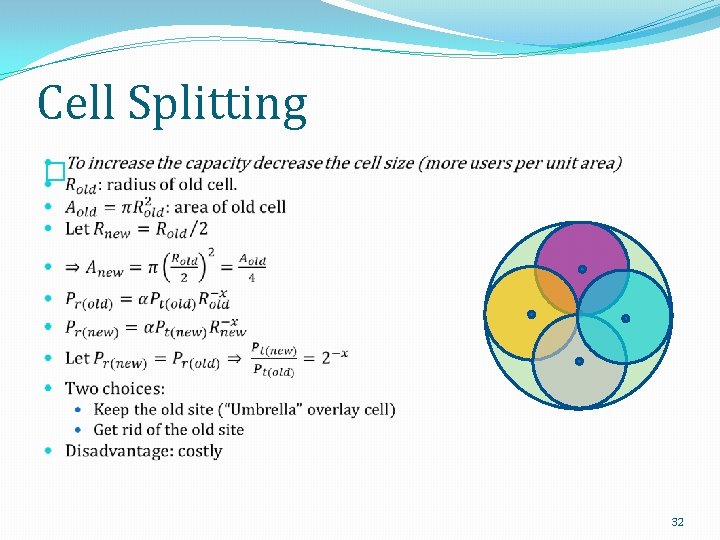
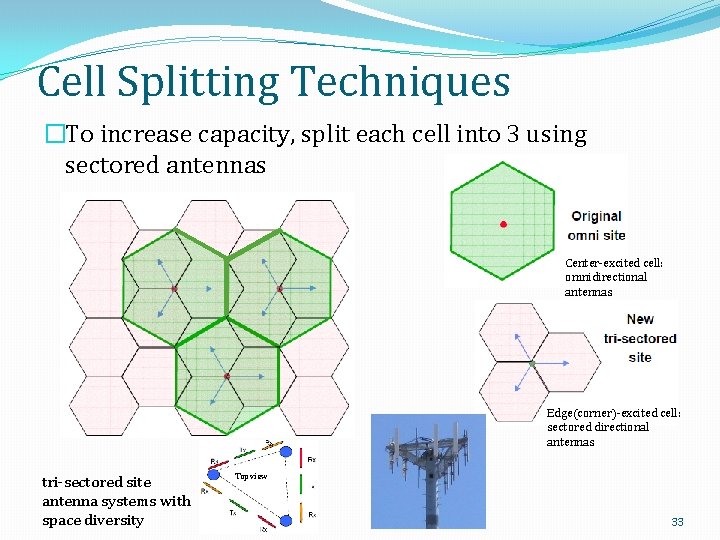
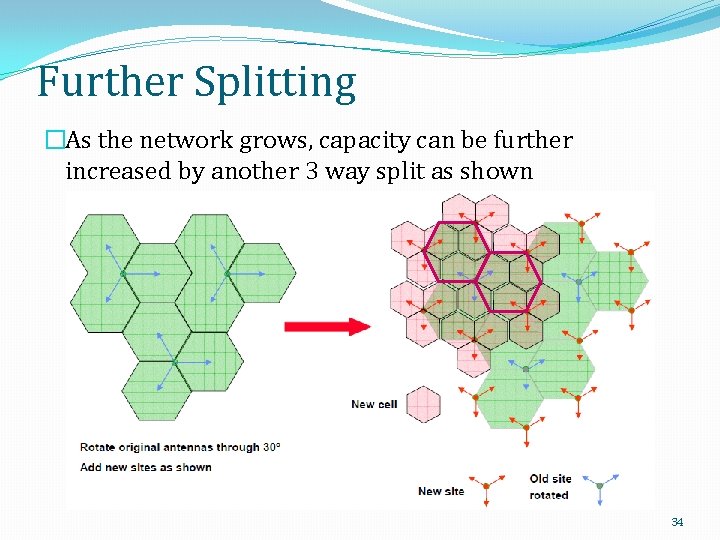
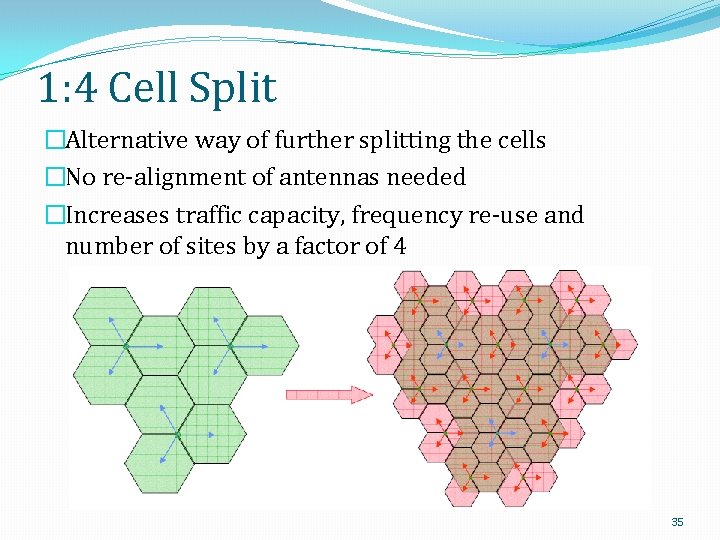
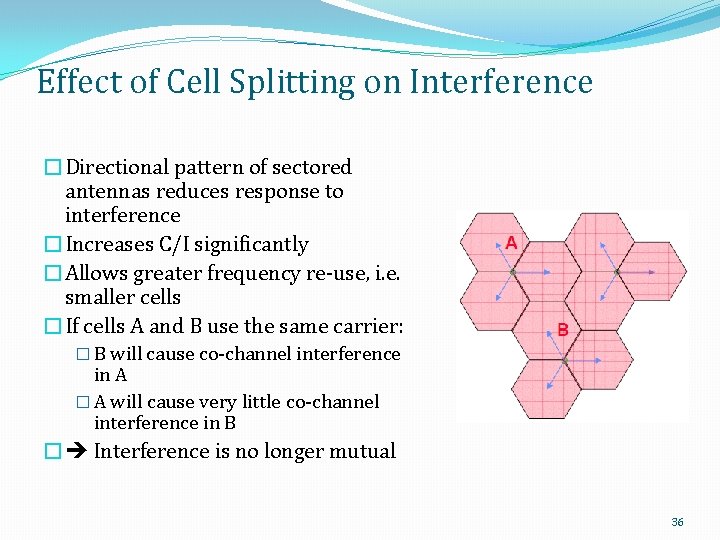
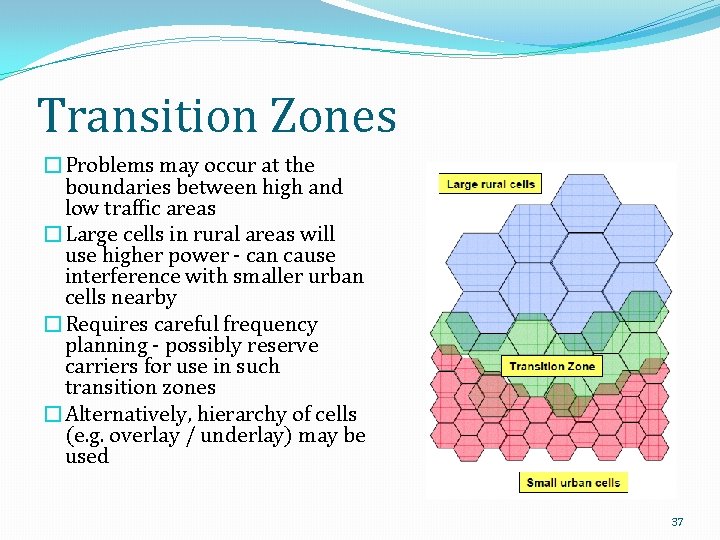
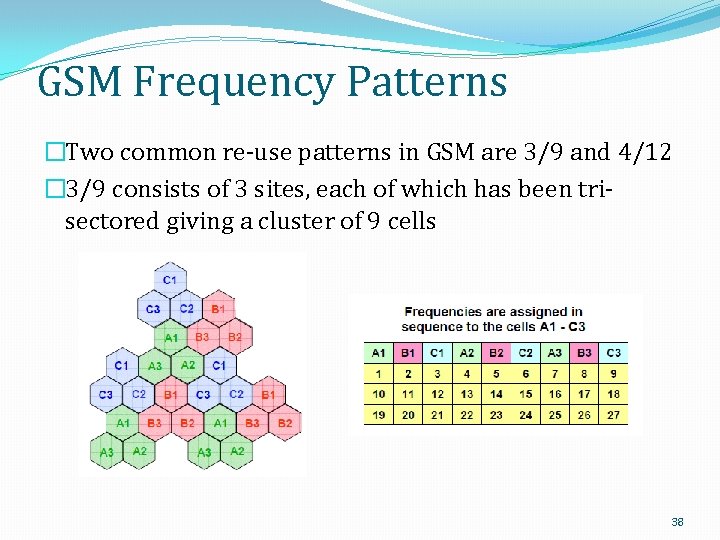
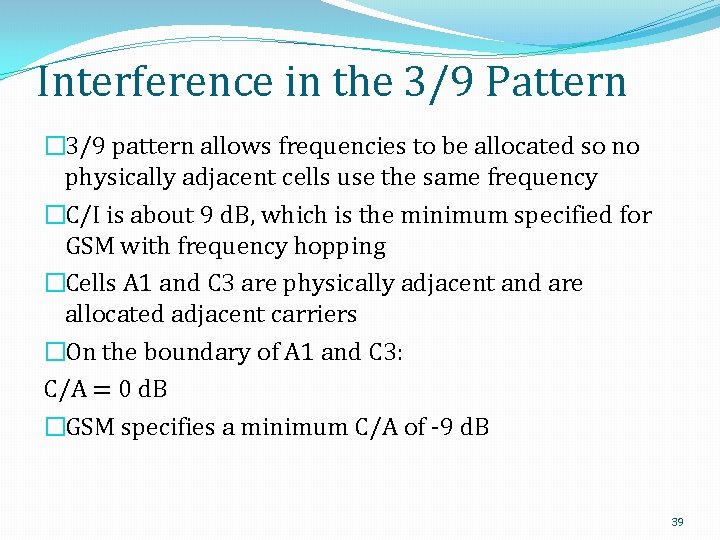
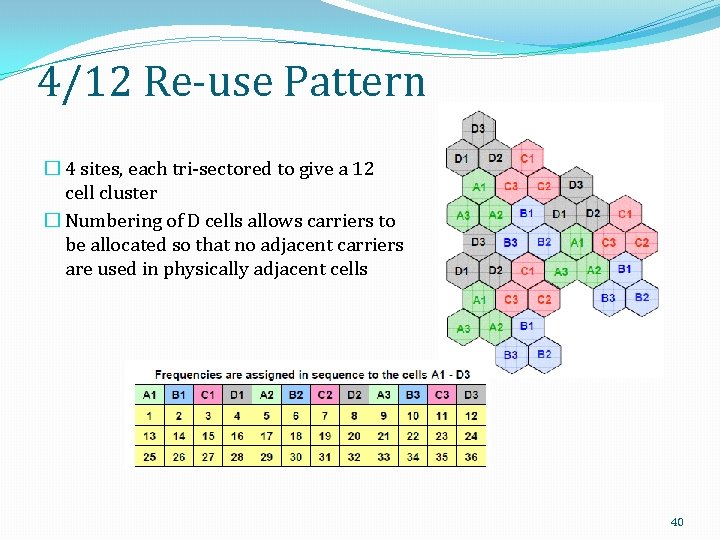
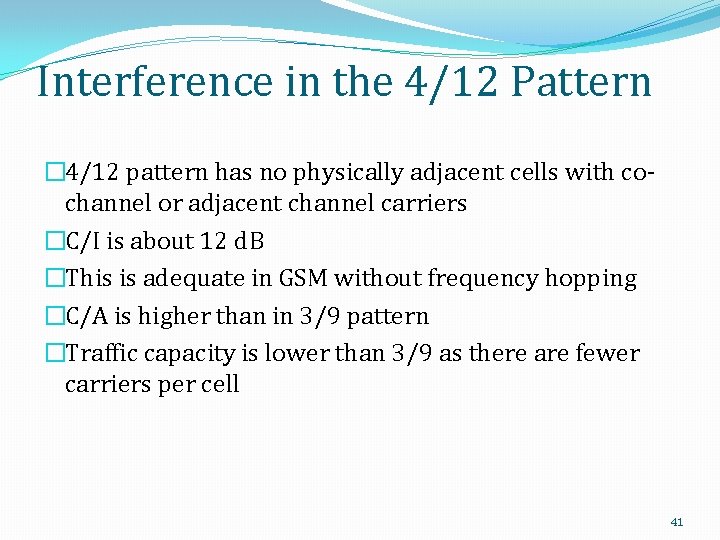
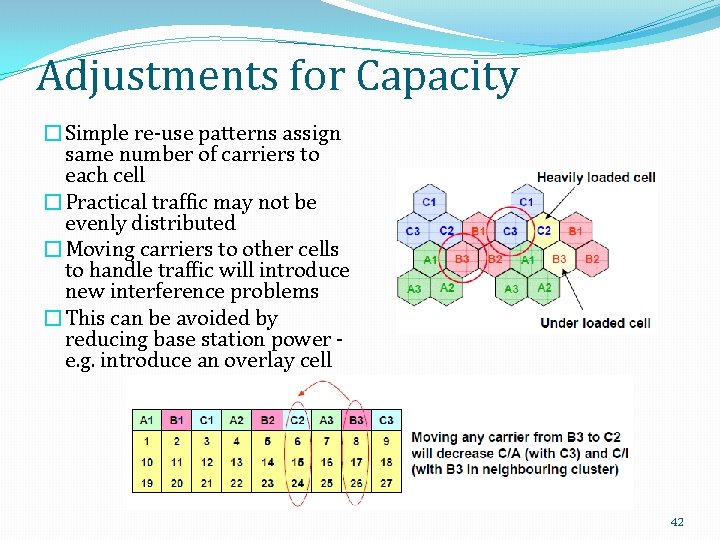
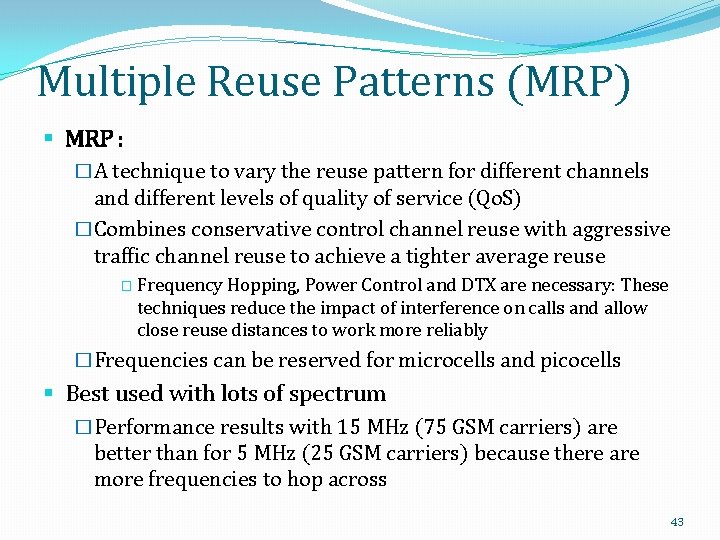
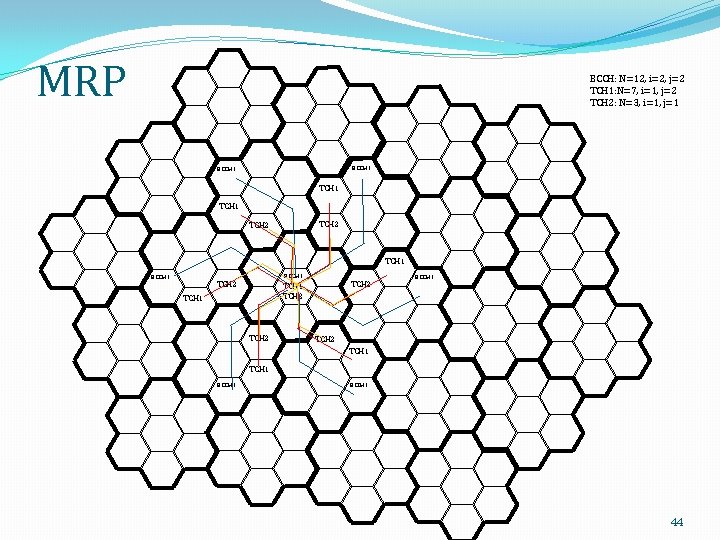
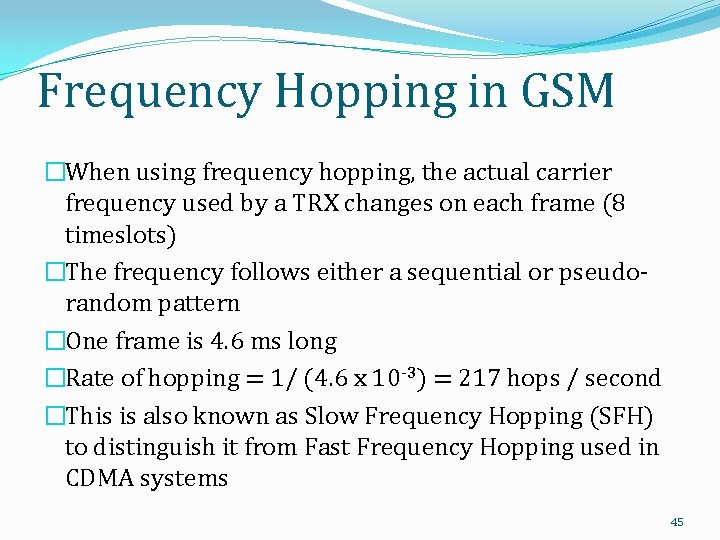
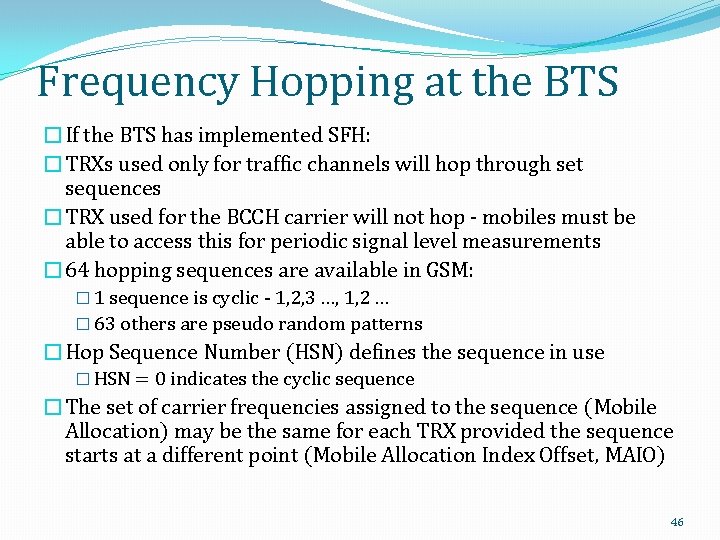
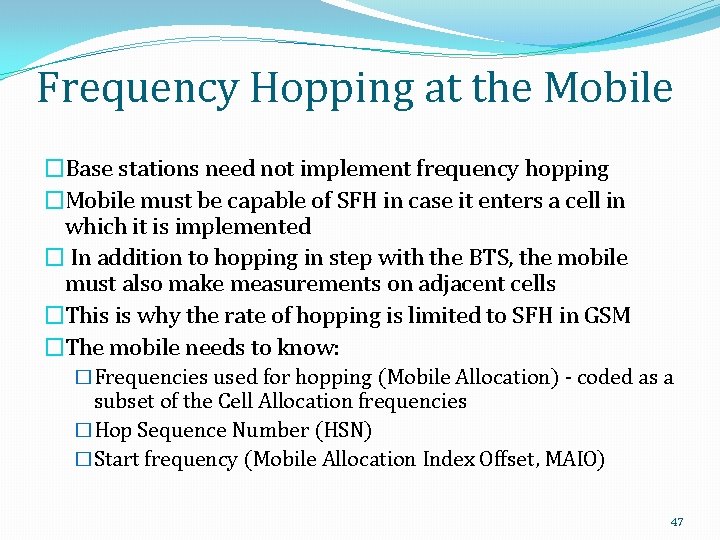
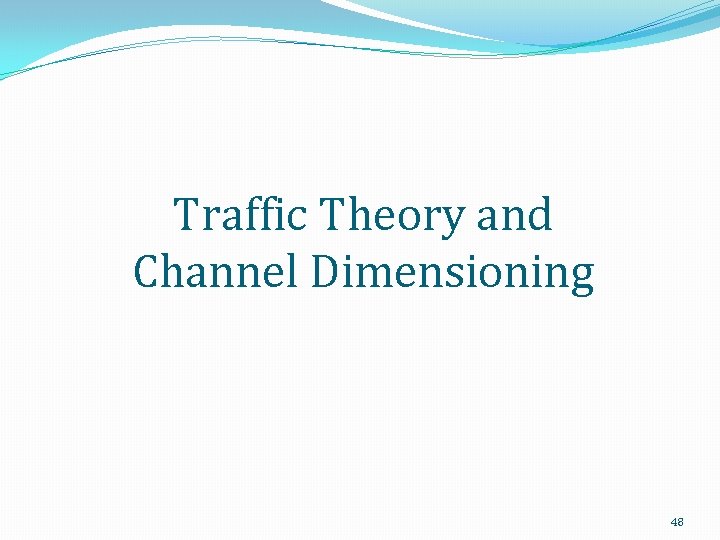
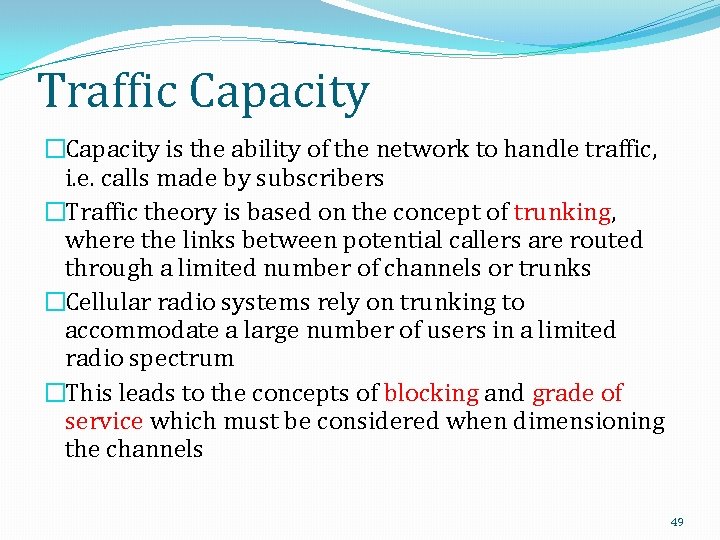
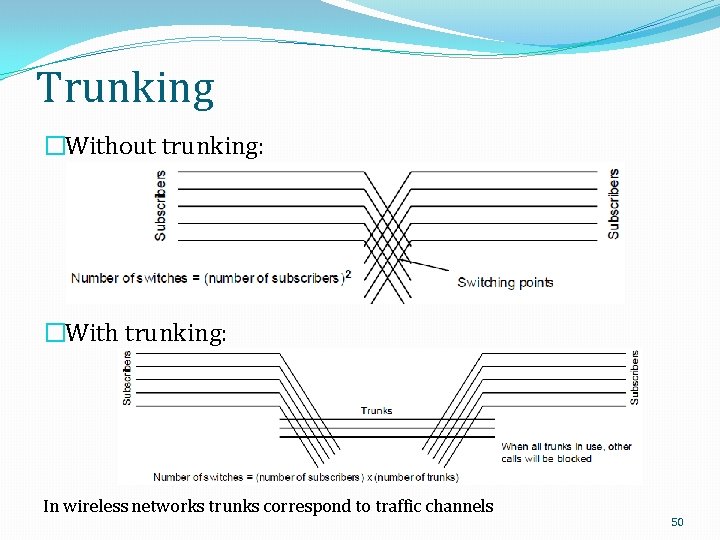
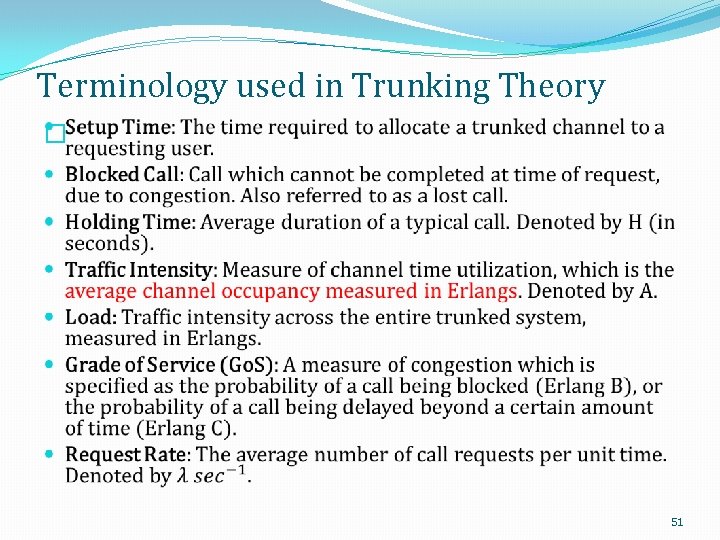
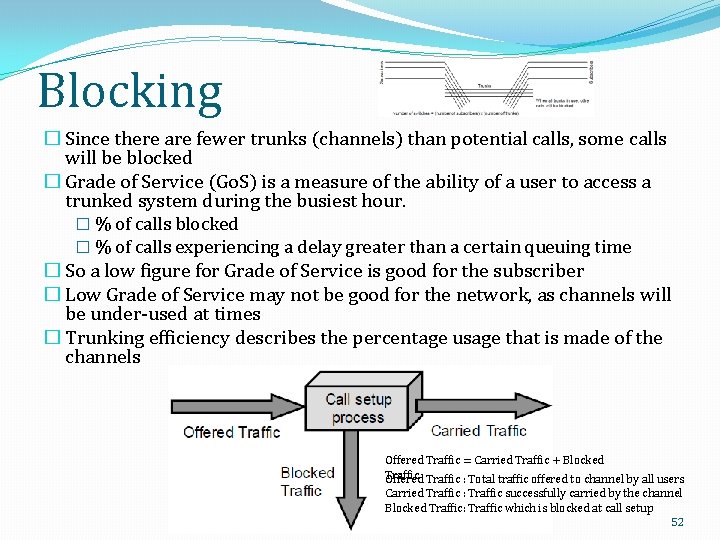
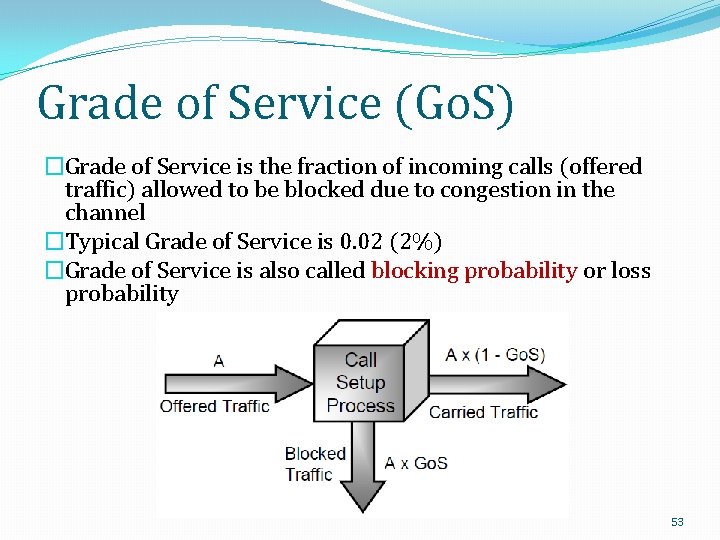
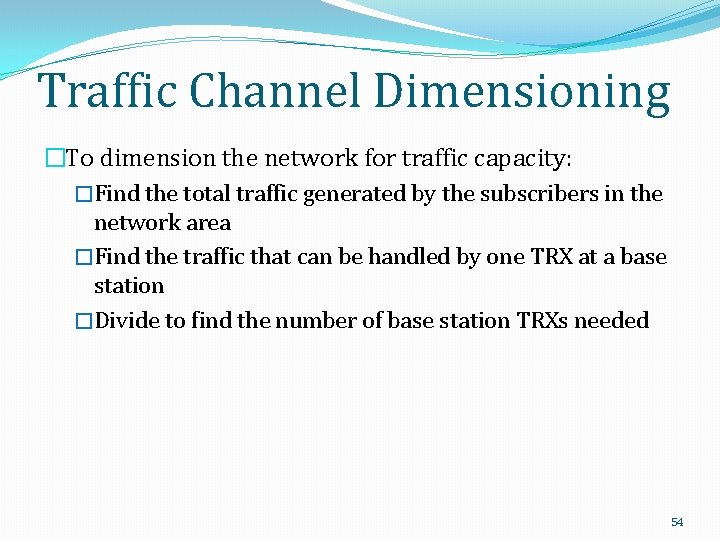
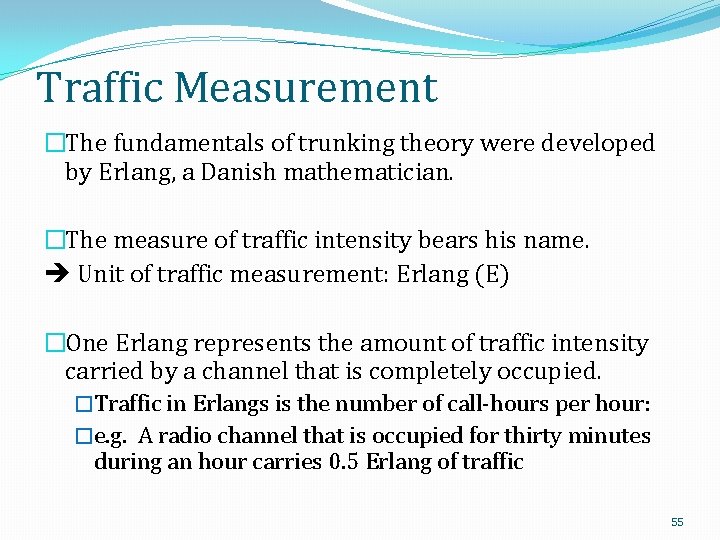
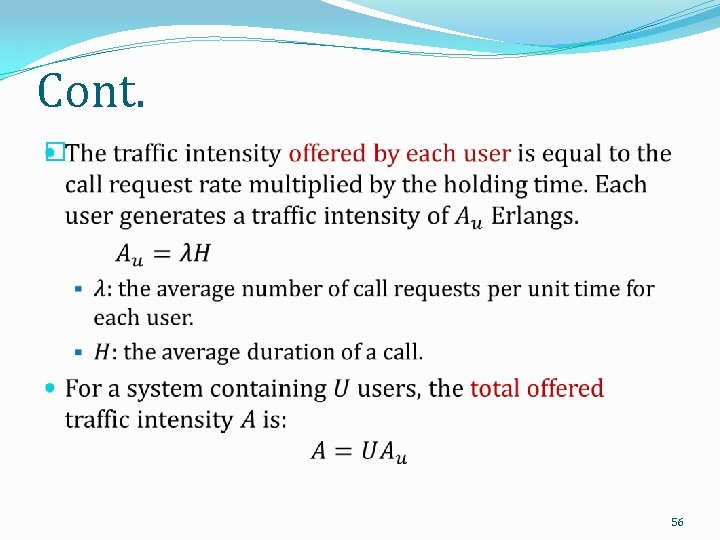
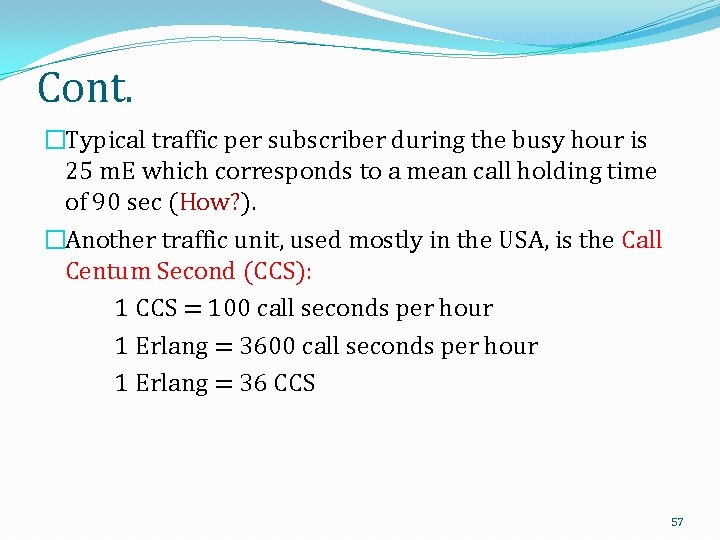
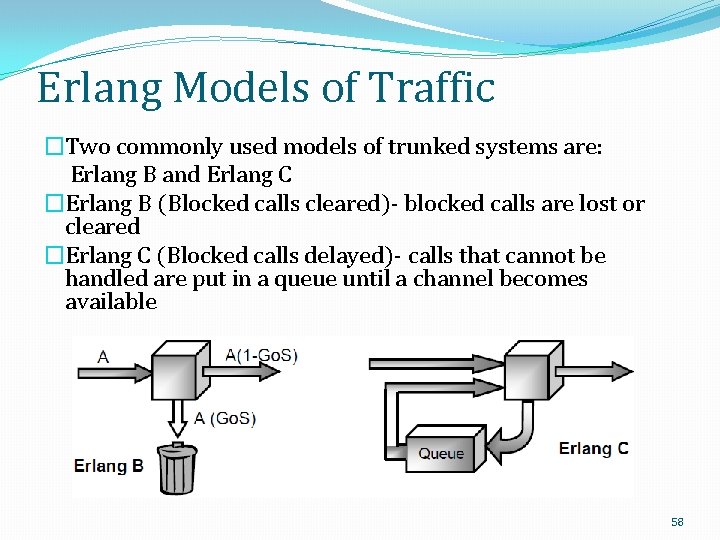
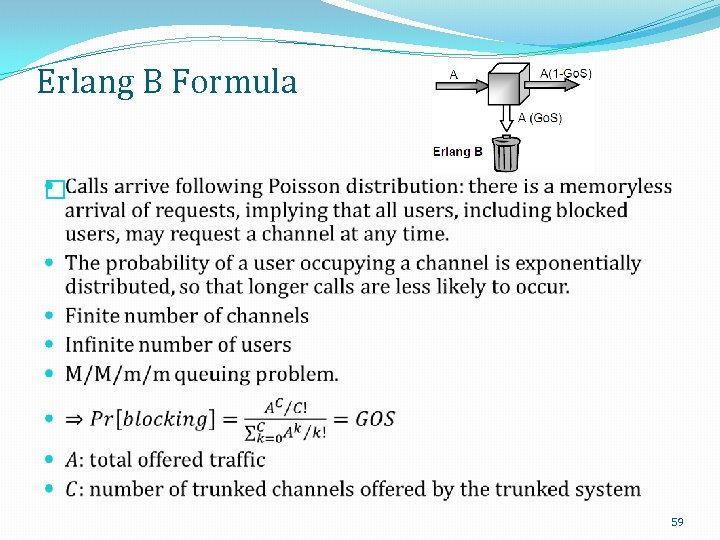
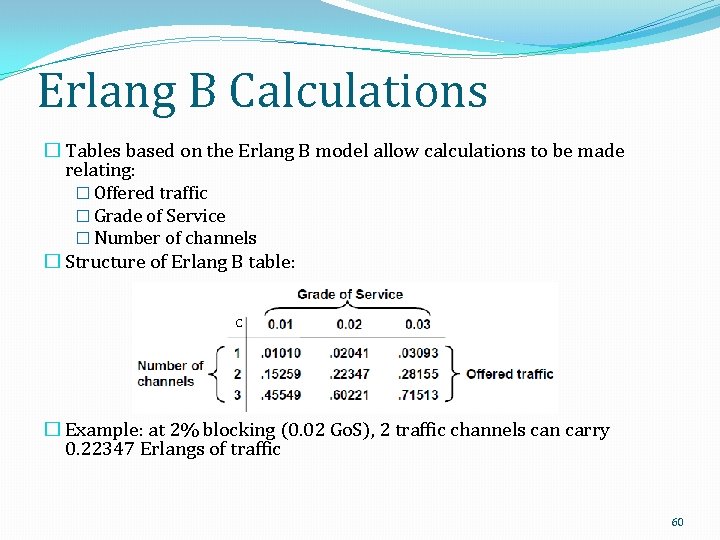
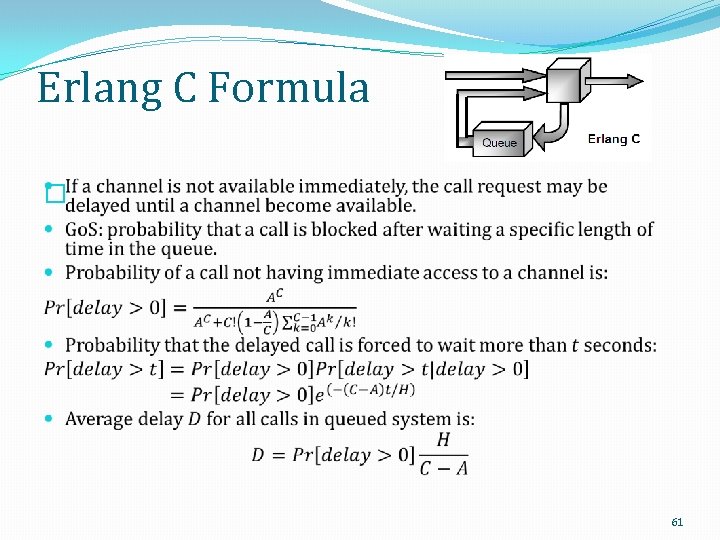
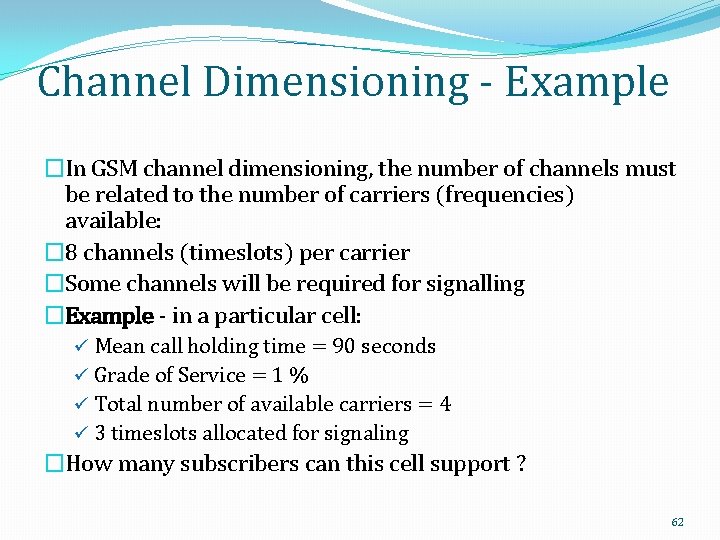
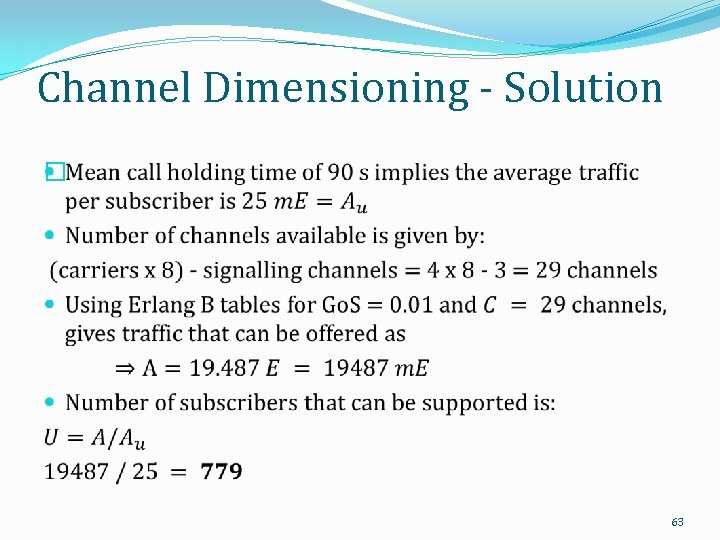
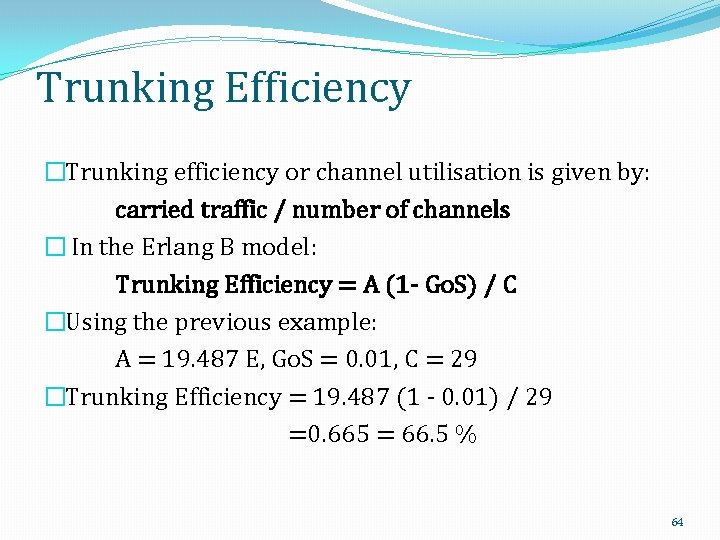
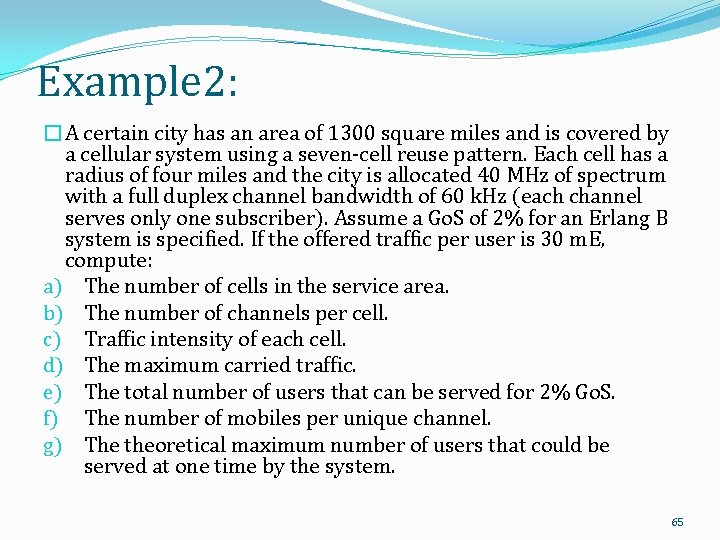
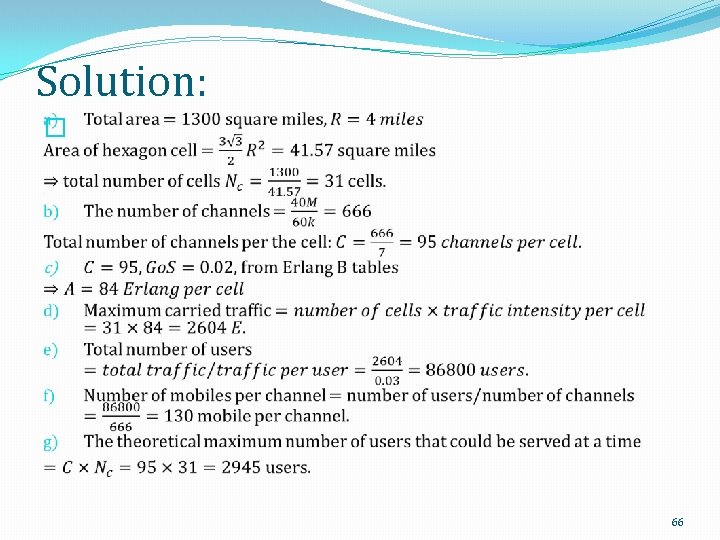
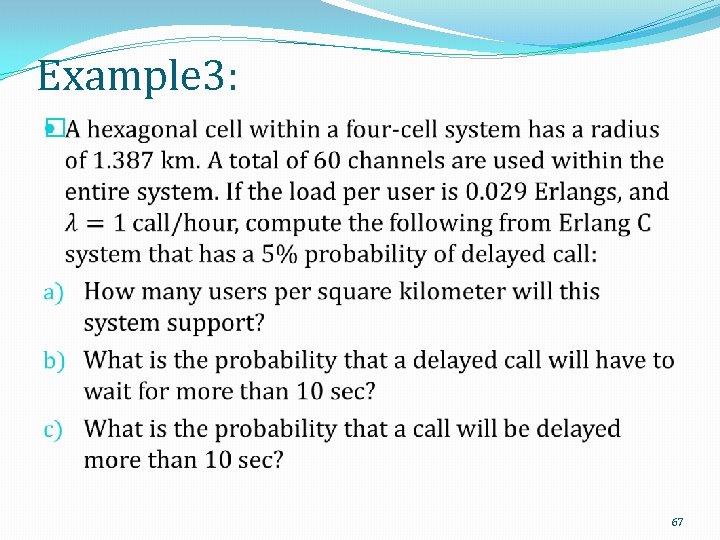
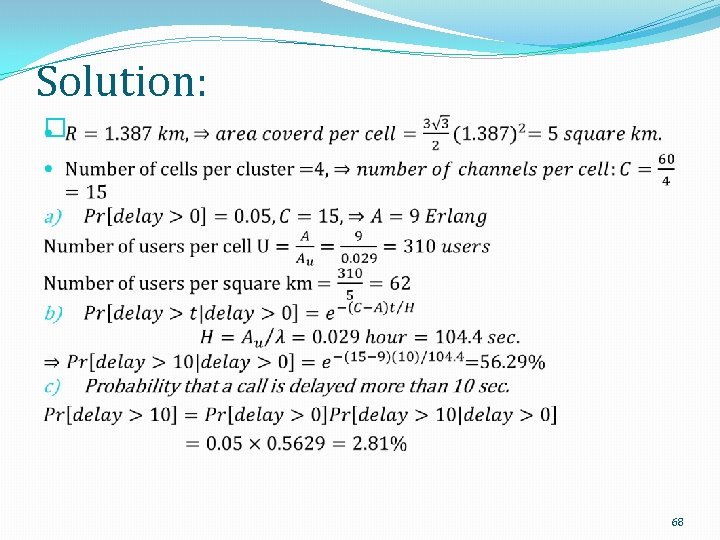
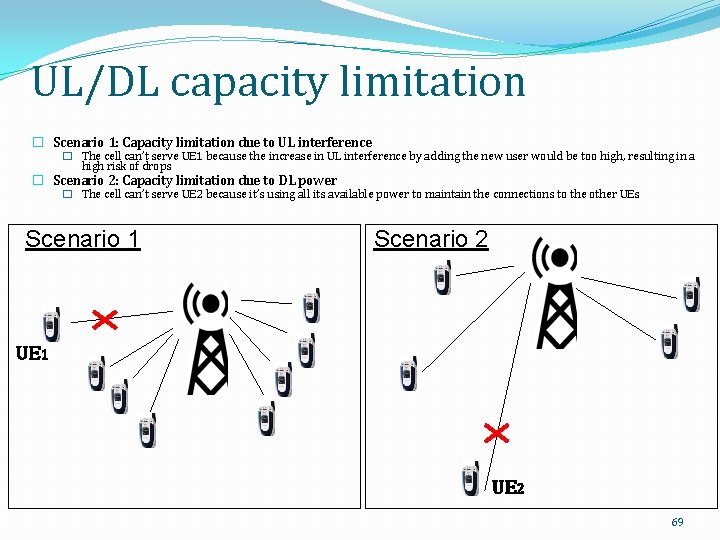
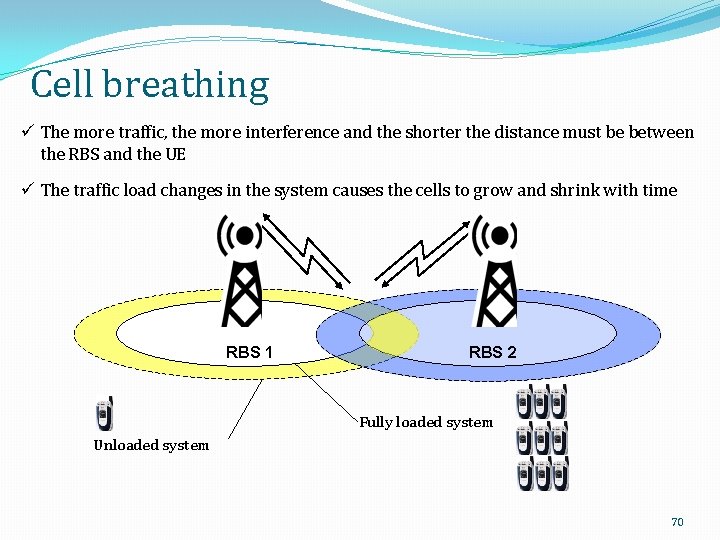
- Slides: 70
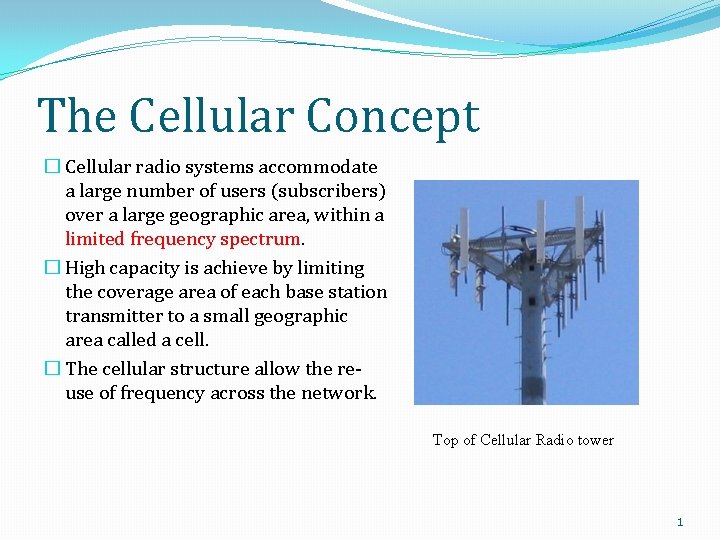
The Cellular Concept � Cellular radio systems accommodate a large number of users (subscribers) over a large geographic area, within a limited frequency spectrum. � High capacity is achieve by limiting the coverage area of each base station transmitter to a small geographic area called a cell. � The cellular structure allow the reuse of frequency across the network. Top of Cellular Radio tower 1
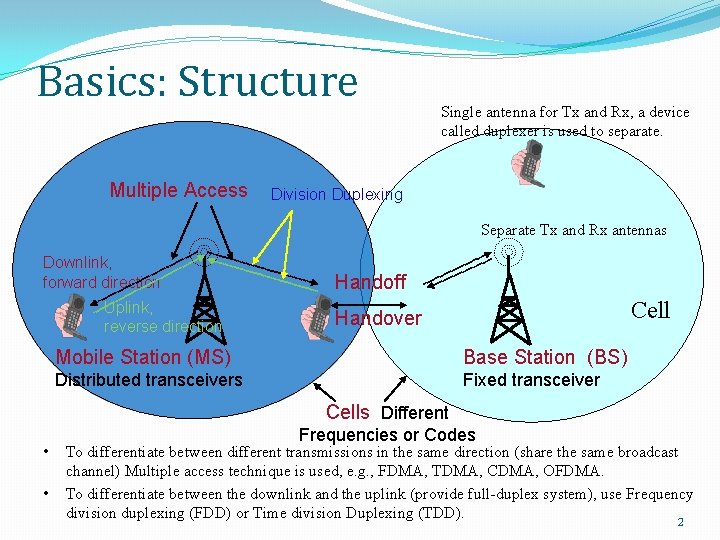
Basics: Structure Multiple Access Single antenna for Tx and Rx, a device called duplexer is used to separate. Division Duplexing Separate Tx and Rx antennas Downlink, forward direction Uplink, reverse direction Handoff Cell Handover Mobile Station (MS) Base Station (BS) Distributed transceivers Fixed transceiver Cells Different • • Frequencies or Codes To differentiate between different transmissions in the same direction (share the same broadcast channel) Multiple access technique is used, e. g. , FDMA, TDMA, CDMA, OFDMA. To differentiate between the downlink and the uplink (provide full-duplex system), use Frequency division duplexing (FDD) or Time division Duplexing (TDD). 2
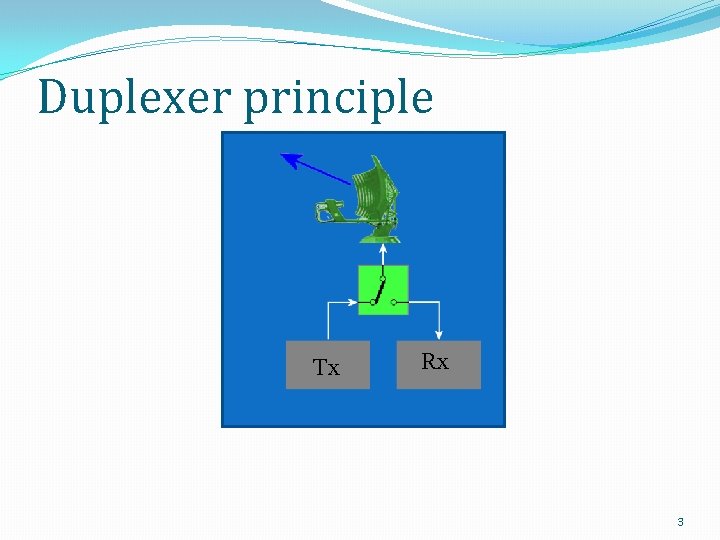
Duplexer principle Tx Rx 3
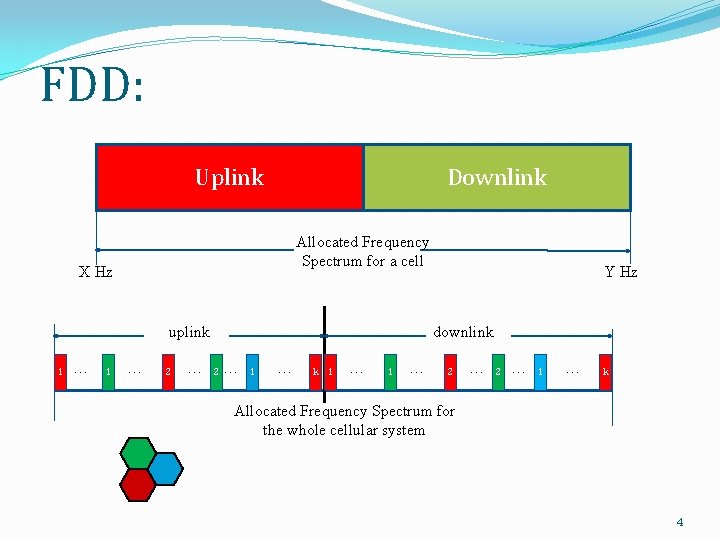
FDD: Uplink Downlink Allocated Frequency Spectrum for a cell X Hz uplink 1 … 2 … Y Hz downlink 2 … 1 … k 1 … 2 … 1 … k Allocated Frequency Spectrum for the whole cellular system 4
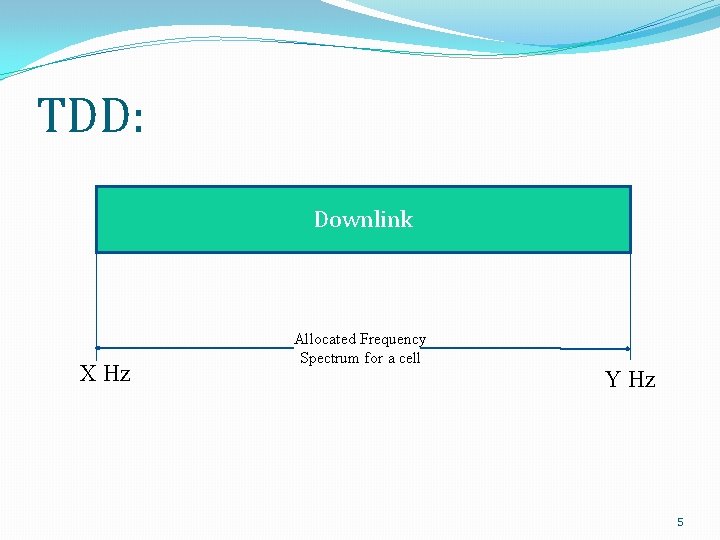
TDD: Downlink Uplink X Hz Allocated Frequency Spectrum for a cell Y Hz 5
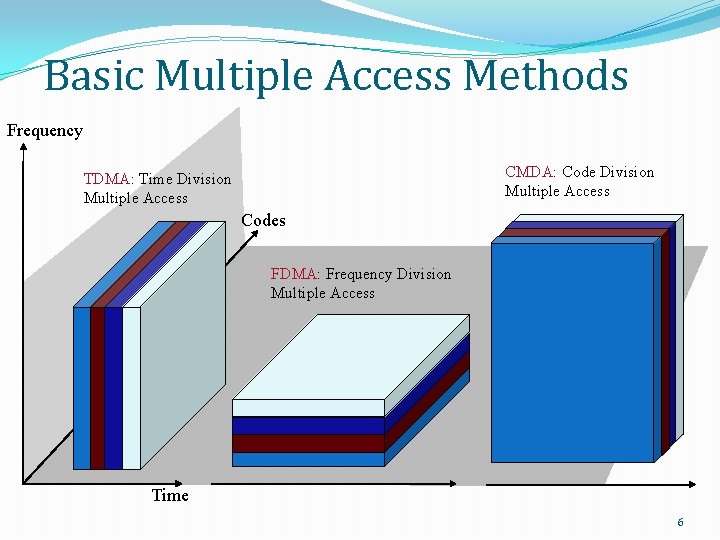
Basic Multiple Access Methods Frequency CMDA: Code Division Multiple Access TDMA: Time Division Multiple Access Codes FDMA: Frequency Division Multiple Access Time 6
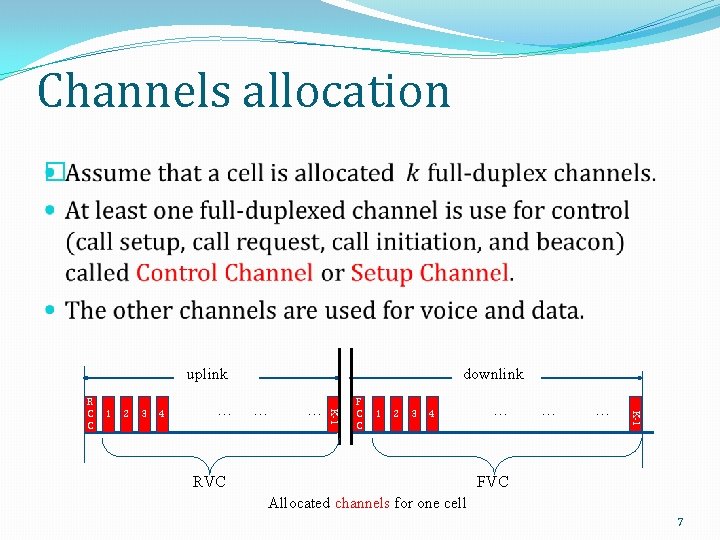
Channels allocation � uplink 2 3 4 … … … F C C 1 2 3 4 RVC … … … K-1 1 K-1 R C C downlink FVC Allocated channels for one cell 7
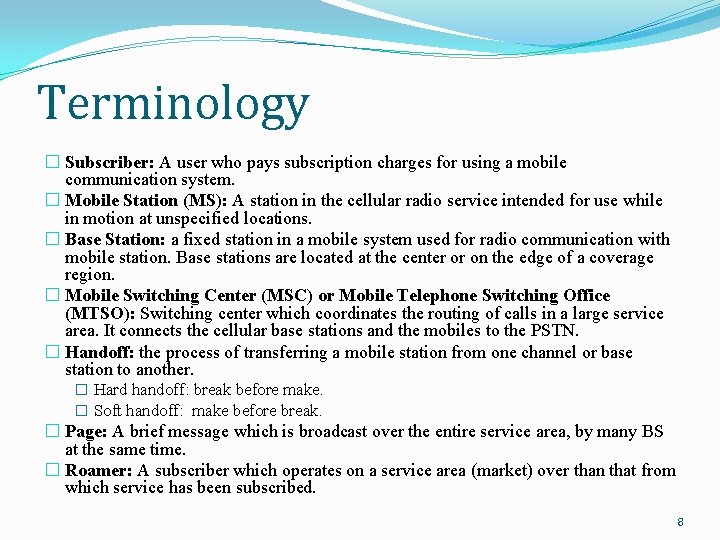
Terminology � Subscriber: A user who pays subscription charges for using a mobile communication system. � Mobile Station (MS): A station in the cellular radio service intended for use while in motion at unspecified locations. � Base Station: a fixed station in a mobile system used for radio communication with mobile station. Base stations are located at the center or on the edge of a coverage region. � Mobile Switching Center (MSC) or Mobile Telephone Switching Office (MTSO): Switching center which coordinates the routing of calls in a large service area. It connects the cellular base stations and the mobiles to the PSTN. � Handoff: the process of transferring a mobile station from one channel or base station to another. � Hard handoff: break before make. � Soft handoff: make before break. � Page: A brief message which is broadcast over the entire service area, by many BS at the same time. � Roamer: A subscriber which operates on a service area (market) over than that from which service has been subscribed. 8
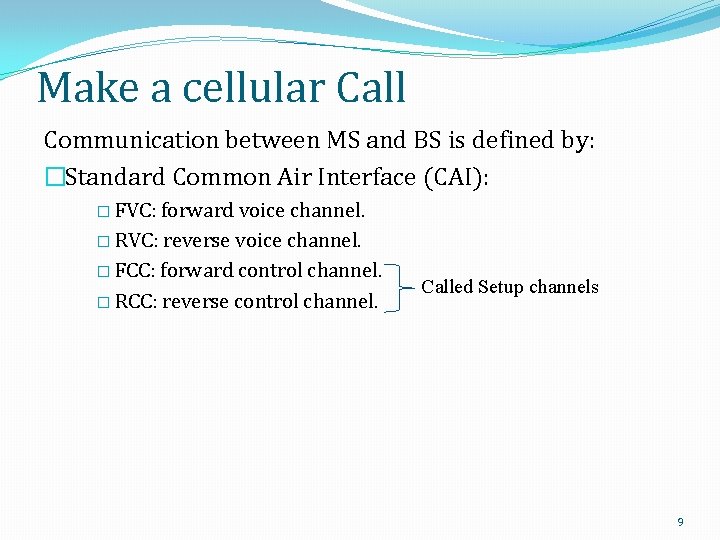
Make a cellular Call Communication between MS and BS is defined by: �Standard Common Air Interface (CAI): � FVC: forward voice channel. � RVC: reverse voice channel. � FCC: forward control channel. � RCC: reverse control channel. Called Setup channels 9
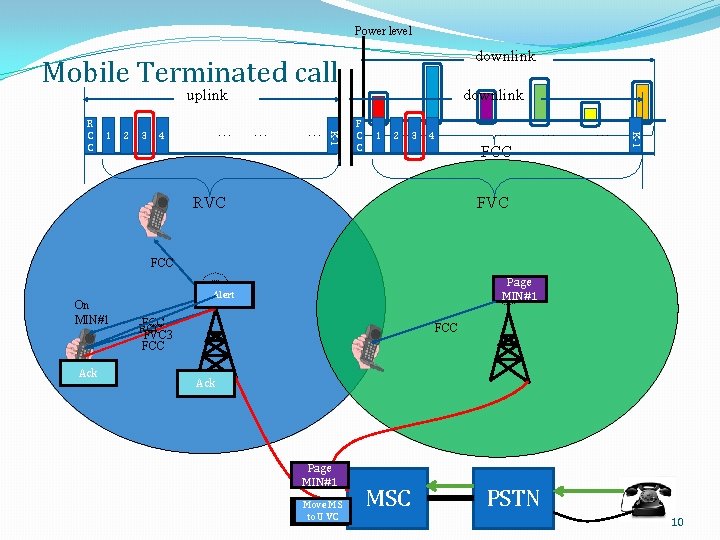
Power level downlink Mobile Terminated call uplink 2 3 … 4 … … F C C 1 2 3 … FCC 4 RVC … … K-1 1 K-1 R C C downlink FVC FCC On MIN#1 Ack Page MIN#1 Page Move MS Alert to U VC MIN#1 FCC RCC FVC 3 FCC Ack Page MIN#1 Move Page. MS to U VC MIN#1 MSC PSTN 10
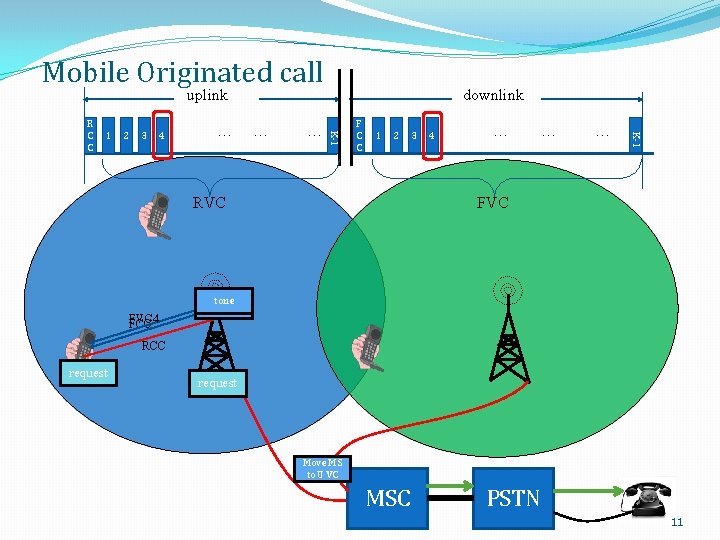
Mobile Originated call uplink 2 3 4 … … … F C C 1 2 RVC FVC 4 FCC 3 4 … … … K-1 1 K-1 R C C downlink FVC Move tone. MS to U VC RCC request Move MS to U VC MSC PSTN 11
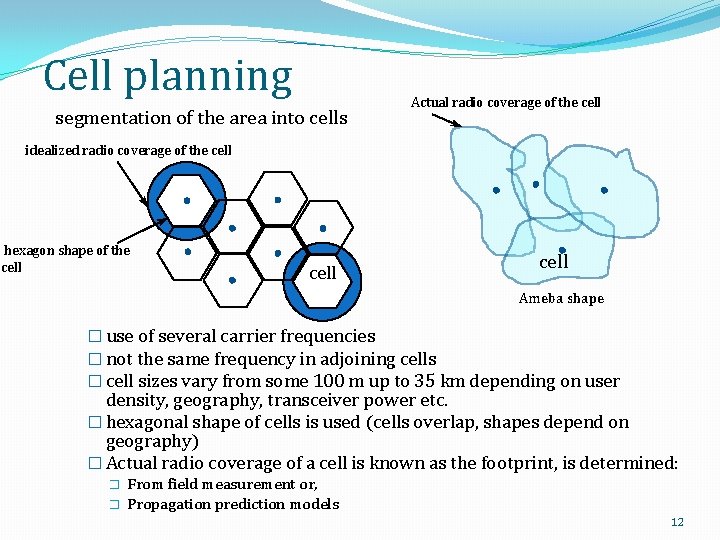
Cell planning segmentation of the area into cells Actual radio coverage of the cell idealized radio coverage of the cell hexagon shape of the cell Ameba shape � use of several carrier frequencies � not the same frequency in adjoining cells � cell sizes vary from some 100 m up to 35 km depending on user density, geography, transceiver power etc. � hexagonal shape of cells is used (cells overlap, shapes depend on geography) � Actual radio coverage of a cell is known as the footprint, is determined: � � From field measurement or, Propagation prediction models 12
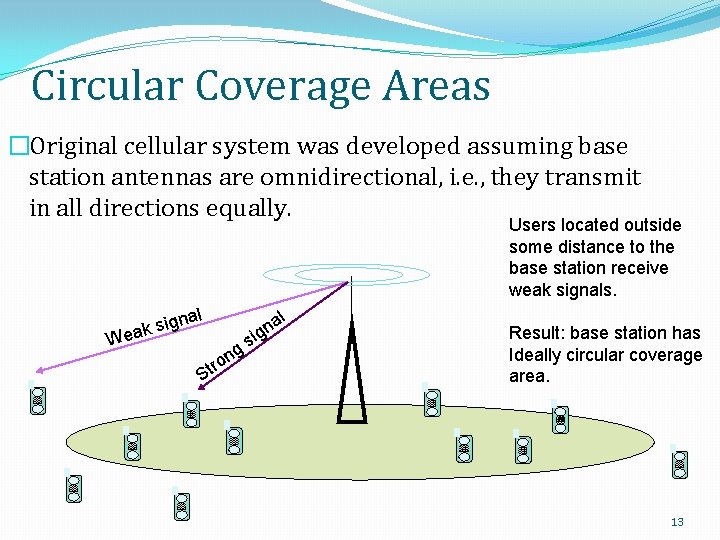
Circular Coverage Areas �Original cellular system was developed assuming base station antennas are omnidirectional, i. e. , they transmit in all directions equally. Users located outside some distance to the base station receive weak signals. al sign k a We S l n tro g na g i s Result: base station has Ideally circular coverage area. 13
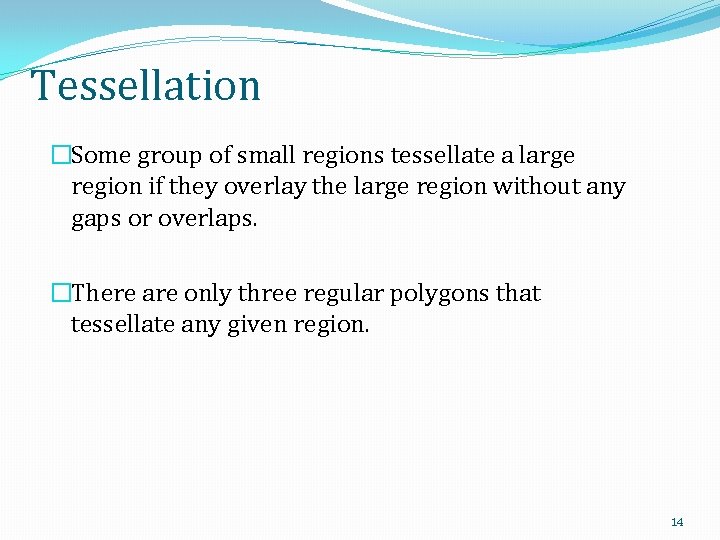
Tessellation �Some group of small regions tessellate a large region if they overlay the large region without any gaps or overlaps. �There are only three regular polygons that tessellate any given region. 14
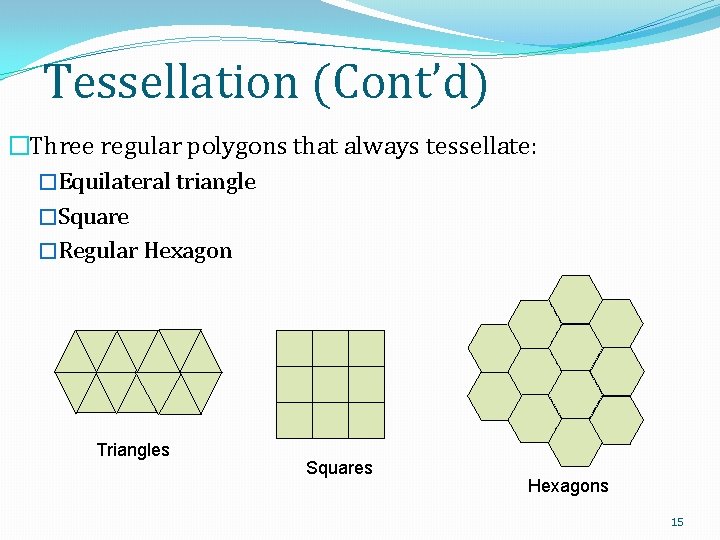
Tessellation (Cont’d) �Three regular polygons that always tessellate: �Equilateral triangle �Square �Regular Hexagon Triangles Squares Hexagons 15
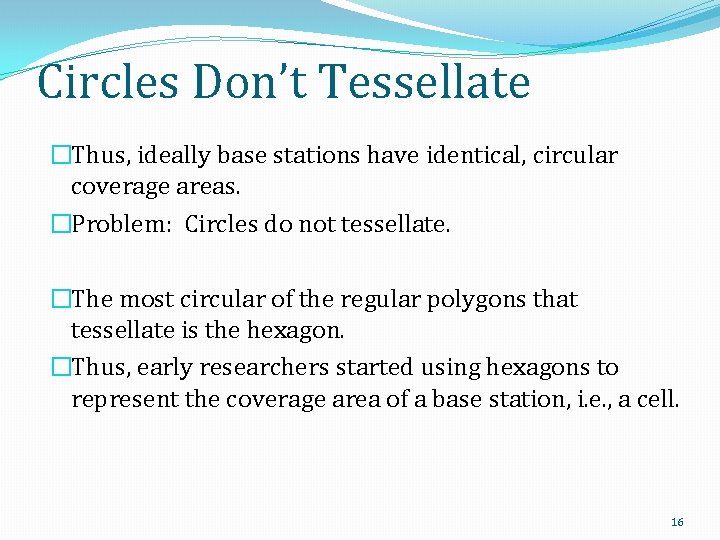
Circles Don’t Tessellate �Thus, ideally base stations have identical, circular coverage areas. �Problem: Circles do not tessellate. �The most circular of the regular polygons that tessellate is the hexagon. �Thus, early researchers started using hexagons to represent the coverage area of a base station, i. e. , a cell. 16
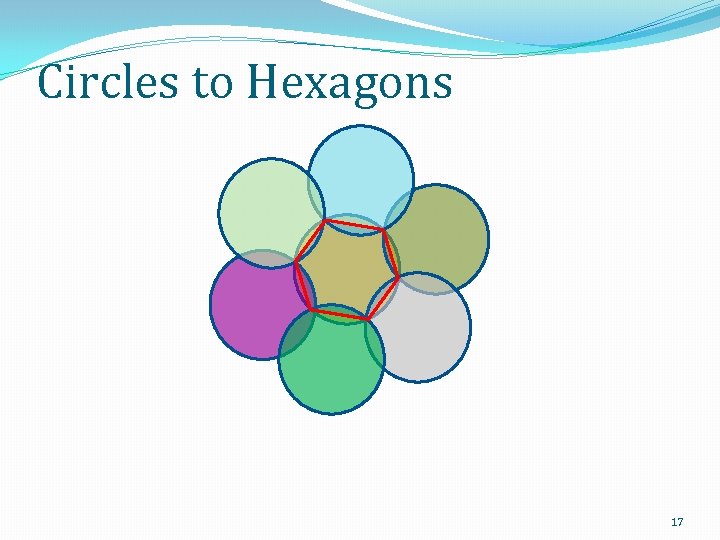
Circles to Hexagons 17
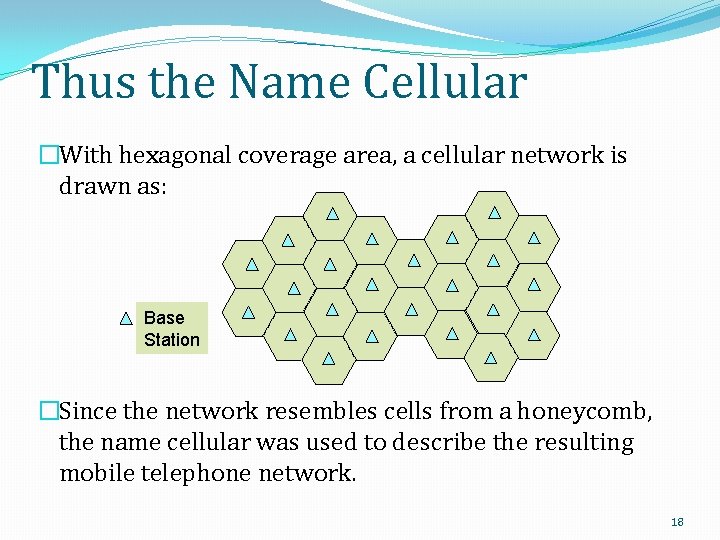
Thus the Name Cellular �With hexagonal coverage area, a cellular network is drawn as: Base Station �Since the network resembles cells from a honeycomb, the name cellular was used to describe the resulting mobile telephone network. 18
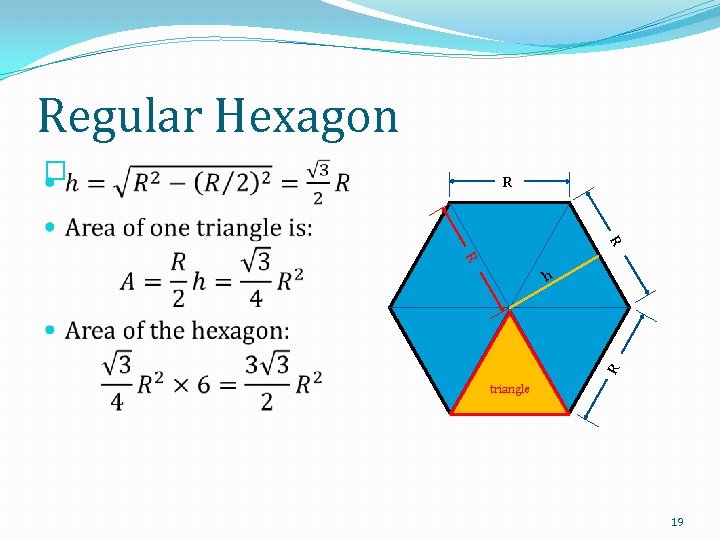
Regular Hexagon � R R h triangle 19
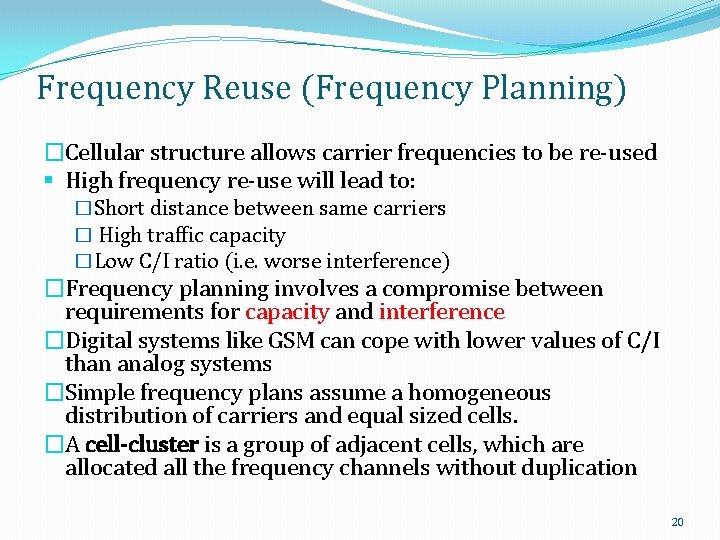
Frequency Reuse (Frequency Planning) �Cellular structure allows carrier frequencies to be re-used § High frequency re-use will lead to: �Short distance between same carriers � High traffic capacity �Low C/I ratio (i. e. worse interference) �Frequency planning involves a compromise between requirements for capacity and interference �Digital systems like GSM can cope with lower values of C/I than analog systems �Simple frequency plans assume a homogeneous distribution of carriers and equal sized cells. �A cell-cluster is a group of adjacent cells, which are allocated all the frequency channels without duplication 20
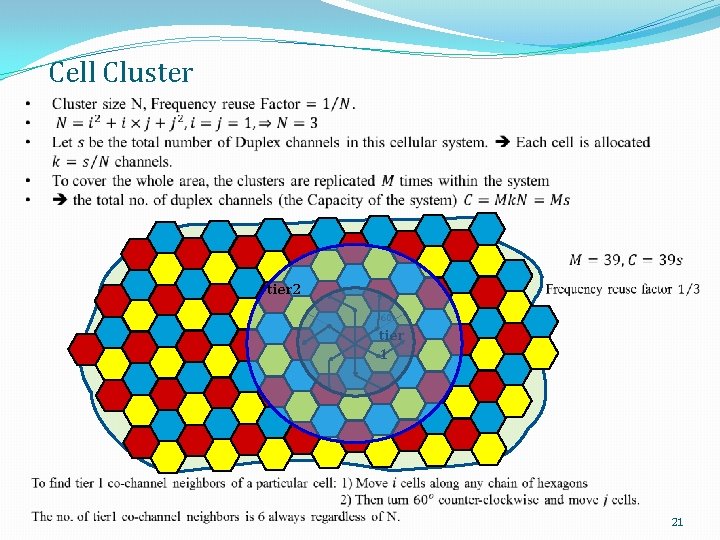
Cell Cluster tier 2 tier 1 21
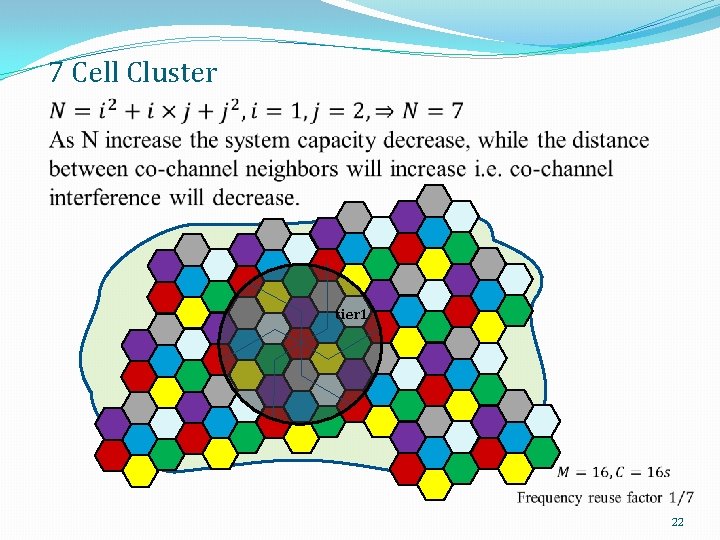
7 Cell Cluster tier 1 22
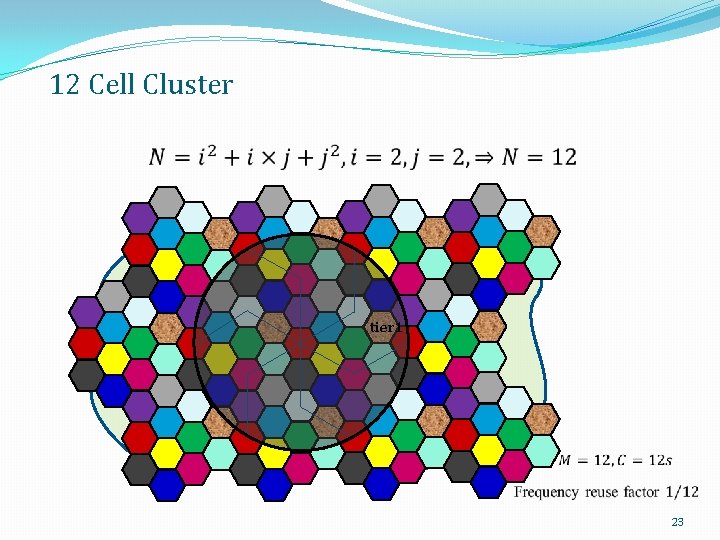
12 Cell Cluster tier 1 23
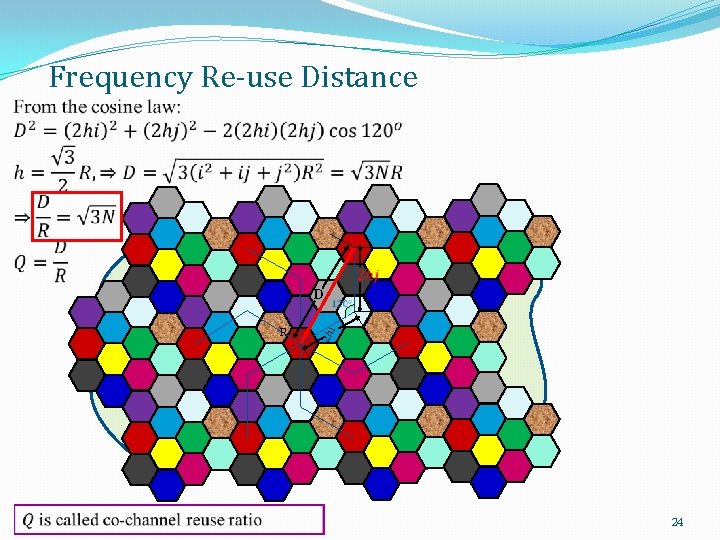
Frequency Re-use Distance D R 24
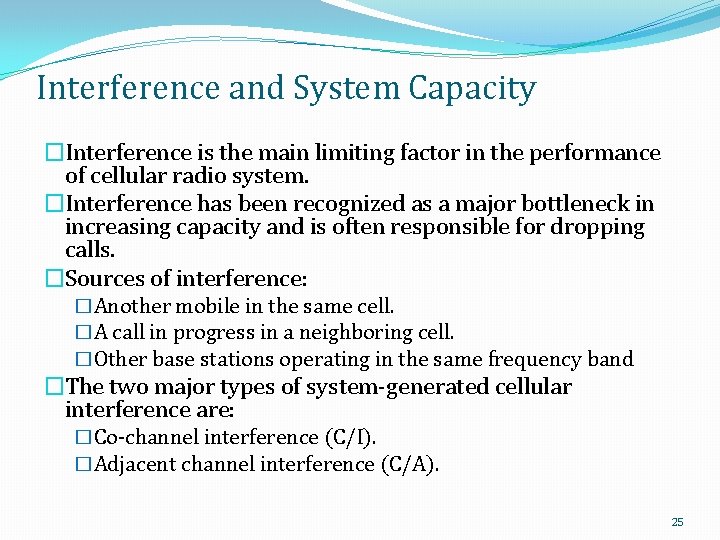
Interference and System Capacity �Interference is the main limiting factor in the performance of cellular radio system. �Interference has been recognized as a major bottleneck in increasing capacity and is often responsible for dropping calls. �Sources of interference: �Another mobile in the same cell. �A call in progress in a neighboring cell. �Other base stations operating in the same frequency band �The two major types of system-generated cellular interference are: �Co-channel interference (C/I). �Adjacent channel interference (C/A). 25
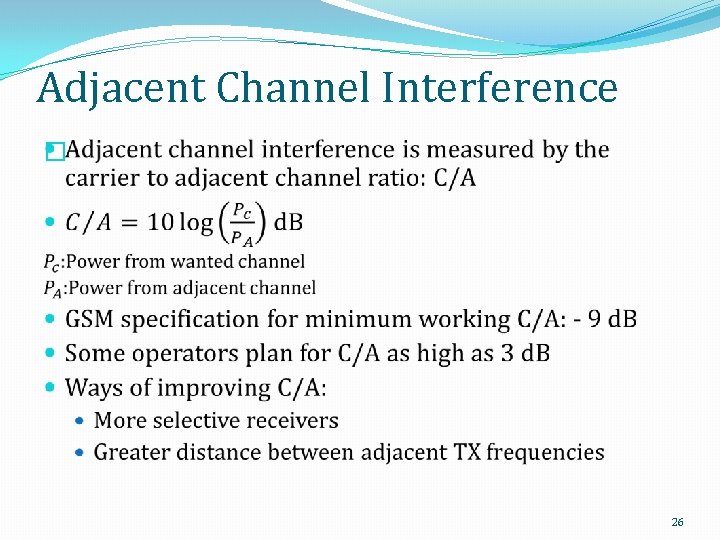
Adjacent Channel Interference � 26
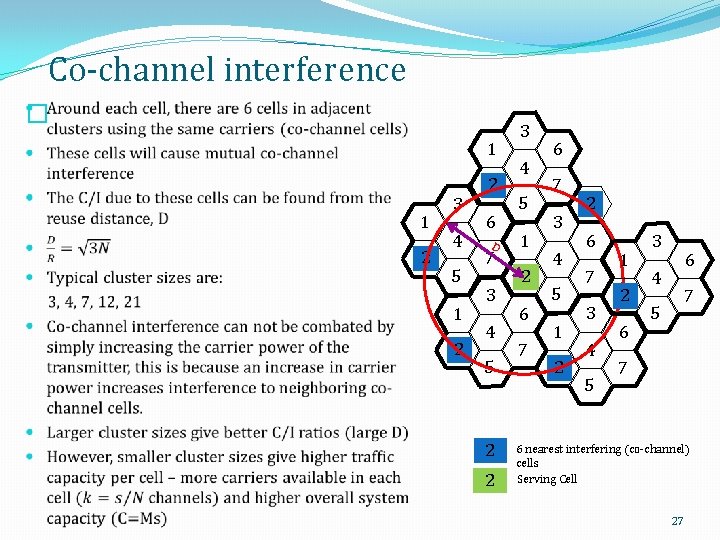
Co-channel interference � 3 1 2 2 4 5 3 1 6 5 D 7 1 2 2 6 4 5 3 1 2 3 6 4 7 7 7 3 1 2 6 4 5 7 6 nearest interfering (co-channel) cells Serving Cell 27
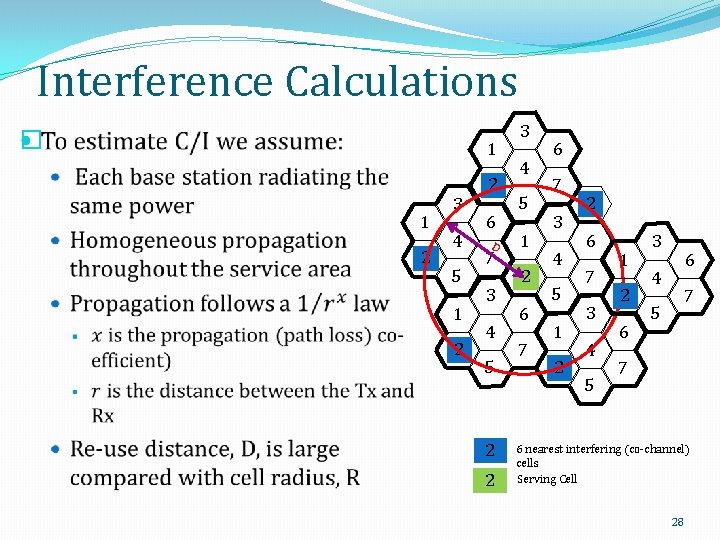
Interference Calculations 3 � 1 2 2 4 5 3 1 6 5 D 7 1 2 2 6 4 5 3 1 2 3 6 4 7 7 7 3 1 2 6 4 5 7 6 nearest interfering (co-channel) cells Serving Cell 28
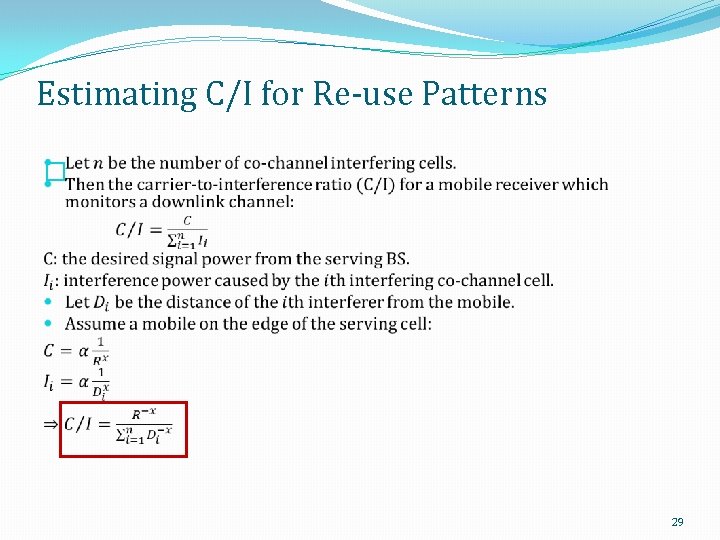
Estimating C/I for Re-use Patterns � 29
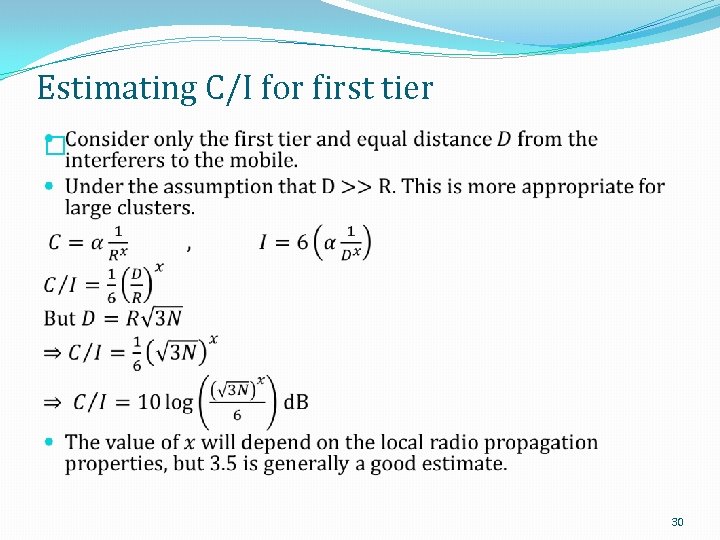
Estimating C/I for first tier � 30
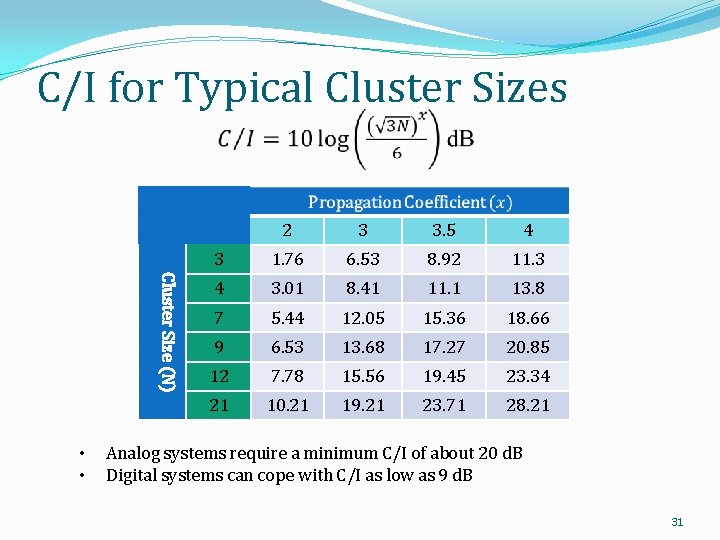
C/I for Typical Cluster Sizes Cluster Size (N) • • 2 3 3. 5 4 3 1. 76 6. 53 8. 92 11. 3 4 3. 01 8. 41 11. 1 13. 8 7 5. 44 12. 05 15. 36 18. 66 9 6. 53 13. 68 17. 27 20. 85 12 7. 78 15. 56 19. 45 23. 34 21 10. 21 19. 21 23. 71 28. 21 Analog systems require a minimum C/I of about 20 d. B Digital systems can cope with C/I as low as 9 d. B 31
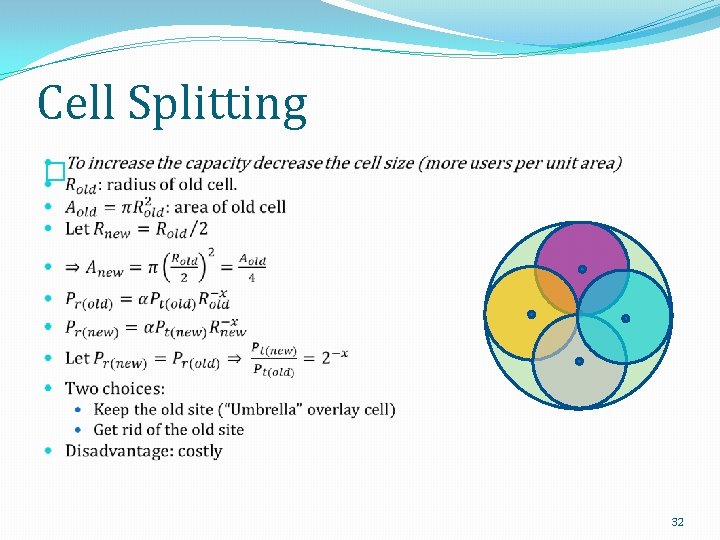
Cell Splitting � 32
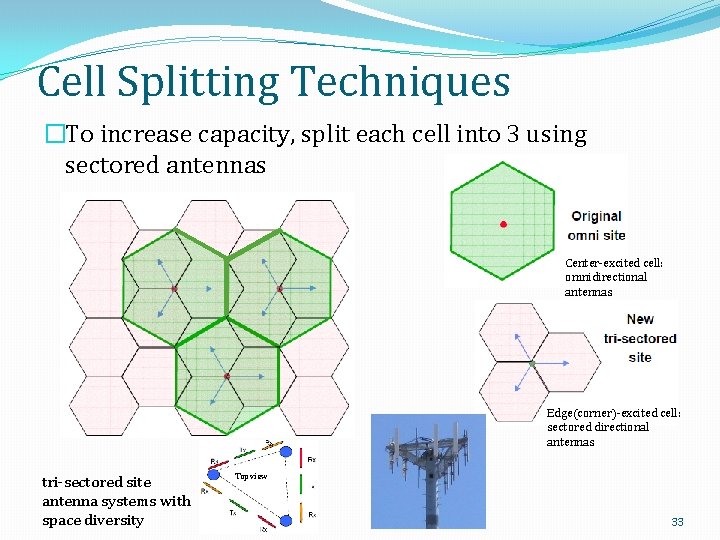
Cell Splitting Techniques �To increase capacity, split each cell into 3 using sectored antennas Center-excited cell: omnidirectional antennas Edge(corner)-excited cell: sectored directional antennas tri-sectored site antenna systems with space diversity Top view 33
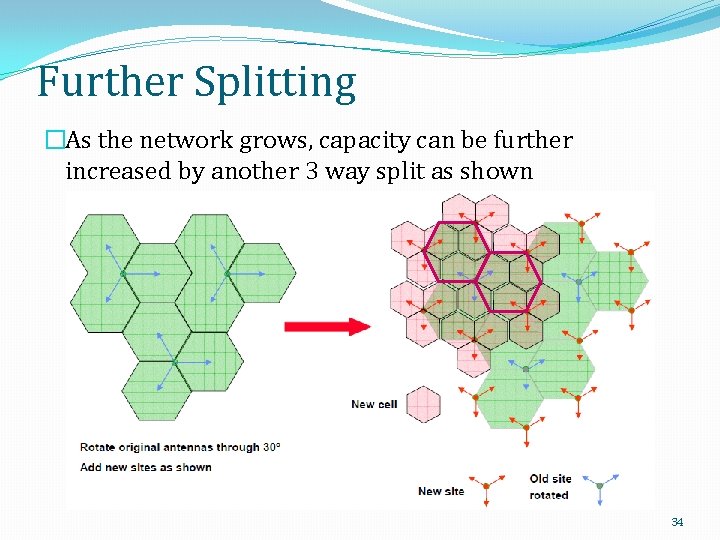
Further Splitting �As the network grows, capacity can be further increased by another 3 way split as shown 34
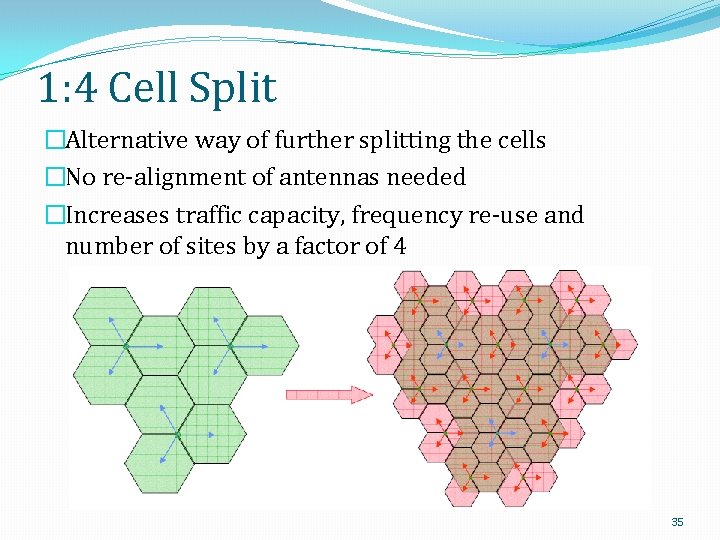
1: 4 Cell Split �Alternative way of further splitting the cells �No re-alignment of antennas needed �Increases traffic capacity, frequency re-use and number of sites by a factor of 4 35
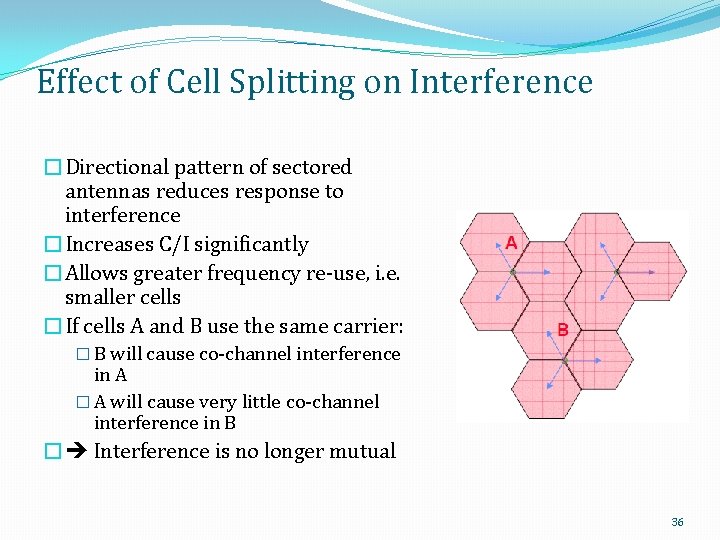
Effect of Cell Splitting on Interference �Directional pattern of sectored antennas reduces response to interference �Increases C/I significantly �Allows greater frequency re-use, i. e. smaller cells �If cells A and B use the same carrier: � B will cause co-channel interference in A � A will cause very little co-channel interference in B � Interference is no longer mutual 36
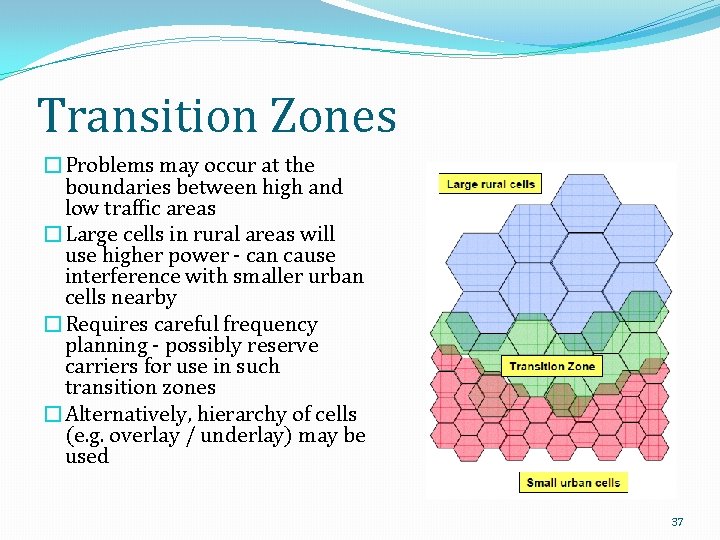
Transition Zones �Problems may occur at the boundaries between high and low traffic areas �Large cells in rural areas will use higher power - can cause interference with smaller urban cells nearby �Requires careful frequency planning - possibly reserve carriers for use in such transition zones �Alternatively, hierarchy of cells (e. g. overlay / underlay) may be used 37
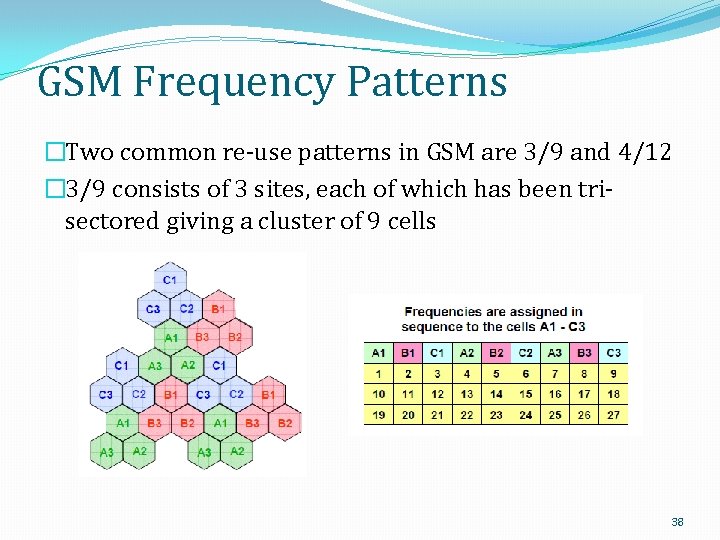
GSM Frequency Patterns �Two common re-use patterns in GSM are 3/9 and 4/12 � 3/9 consists of 3 sites, each of which has been trisectored giving a cluster of 9 cells 38
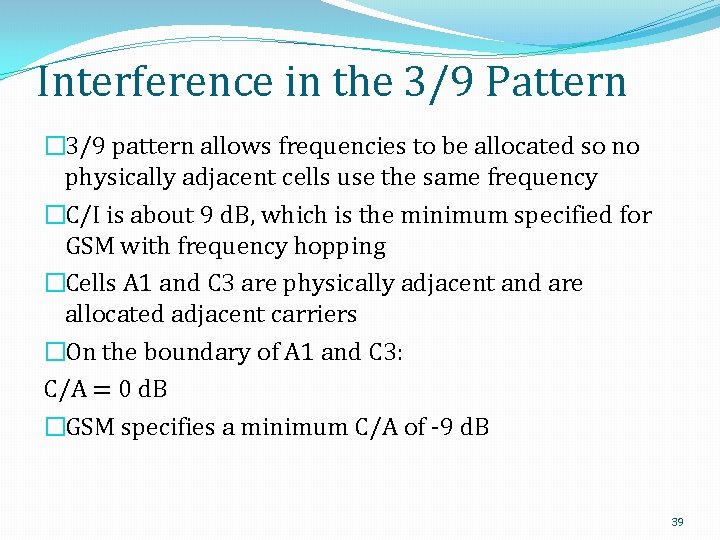
Interference in the 3/9 Pattern � 3/9 pattern allows frequencies to be allocated so no physically adjacent cells use the same frequency �C/I is about 9 d. B, which is the minimum specified for GSM with frequency hopping �Cells A 1 and C 3 are physically adjacent and are allocated adjacent carriers �On the boundary of A 1 and C 3: C/A = 0 d. B �GSM specifies a minimum C/A of -9 d. B 39
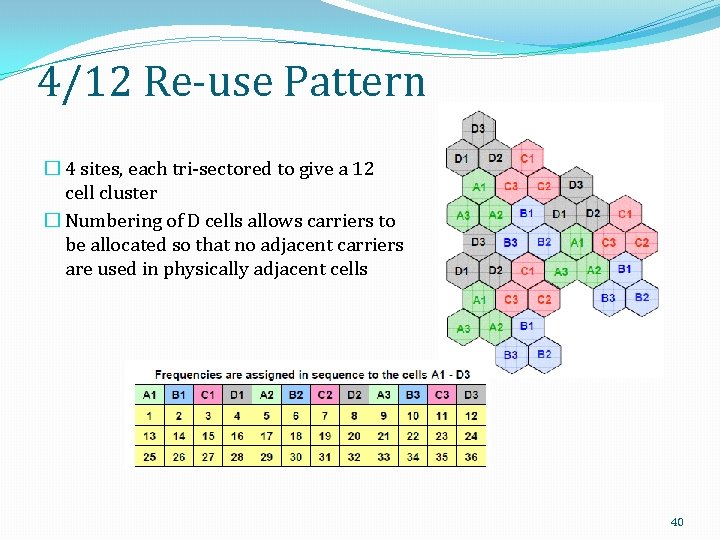
4/12 Re-use Pattern � 4 sites, each tri-sectored to give a 12 cell cluster � Numbering of D cells allows carriers to be allocated so that no adjacent carriers are used in physically adjacent cells 40
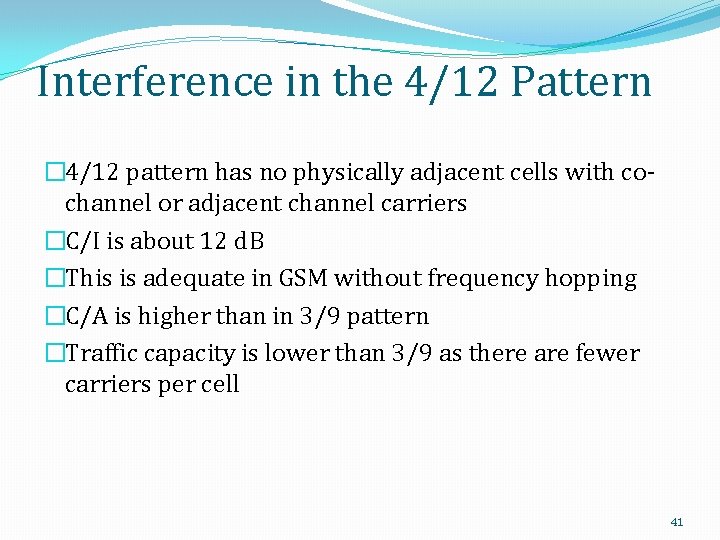
Interference in the 4/12 Pattern � 4/12 pattern has no physically adjacent cells with cochannel or adjacent channel carriers �C/I is about 12 d. B �This is adequate in GSM without frequency hopping �C/A is higher than in 3/9 pattern �Traffic capacity is lower than 3/9 as there are fewer carriers per cell 41
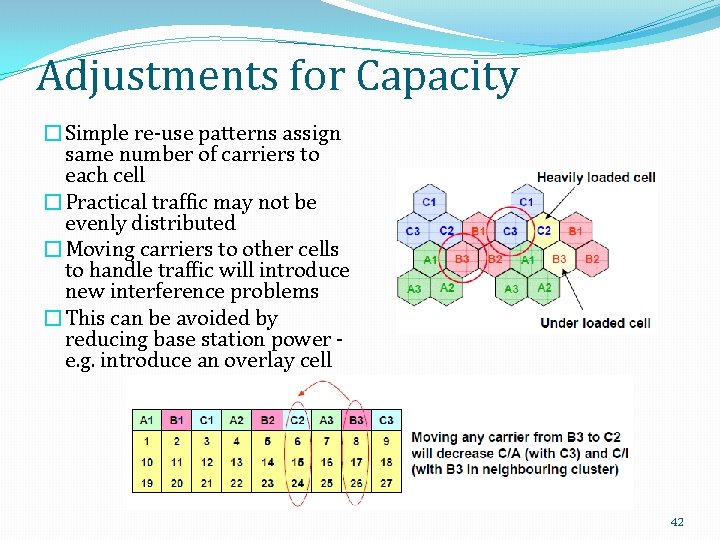
Adjustments for Capacity �Simple re-use patterns assign same number of carriers to each cell �Practical traffic may not be evenly distributed �Moving carriers to other cells to handle traffic will introduce new interference problems �This can be avoided by reducing base station power e. g. introduce an overlay cell 42
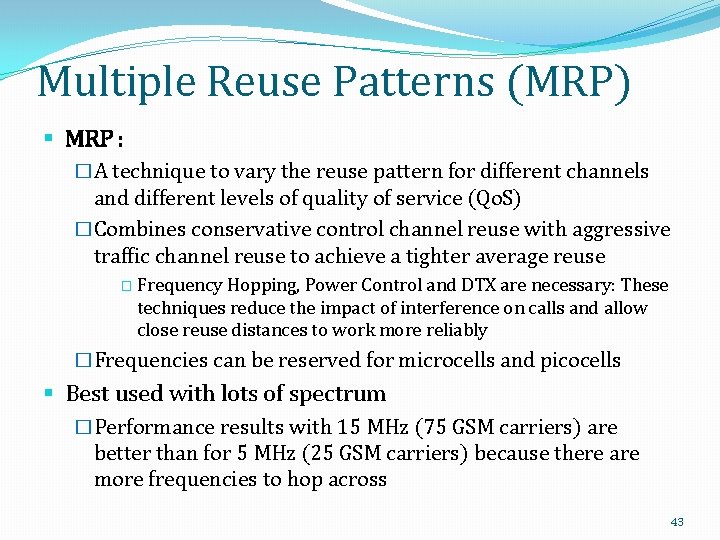
Multiple Reuse Patterns (MRP) § MRP : �A technique to vary the reuse pattern for different channels and different levels of quality of service (Qo. S) �Combines conservative control channel reuse with aggressive traffic channel reuse to achieve a tighter average reuse � Frequency Hopping, Power Control and DTX are necessary: These techniques reduce the impact of interference on calls and allow close reuse distances to work more reliably �Frequencies can be reserved for microcells and picocells § Best used with lots of spectrum �Performance results with 15 MHz (75 GSM carriers) are better than for 5 MHz (25 GSM carriers) because there are more frequencies to hop across 43
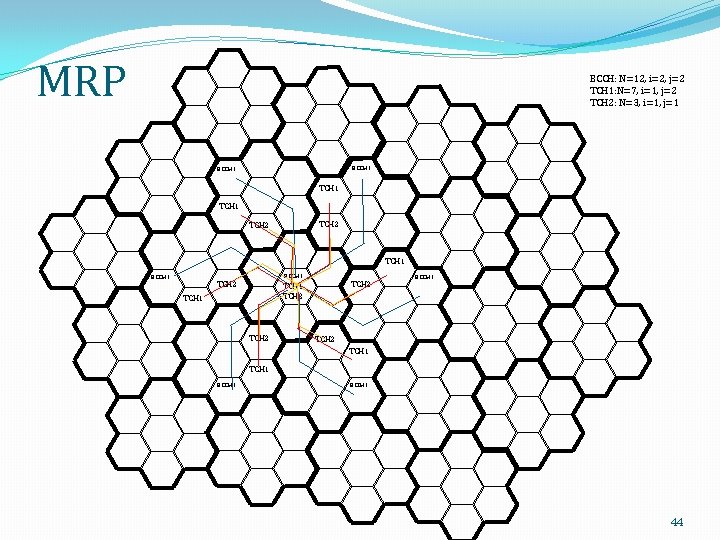
MRP BCCH: N=12, i=2, j=2 TCH 1: N=7, i=1, j=2 TCH 2: N=3, i=1, j=1 BCCH 1 TCH 2 TCH 1 BCCH 1 TCH 2 TCH 1 TCH 2 TCH 1 BCCH 1 44
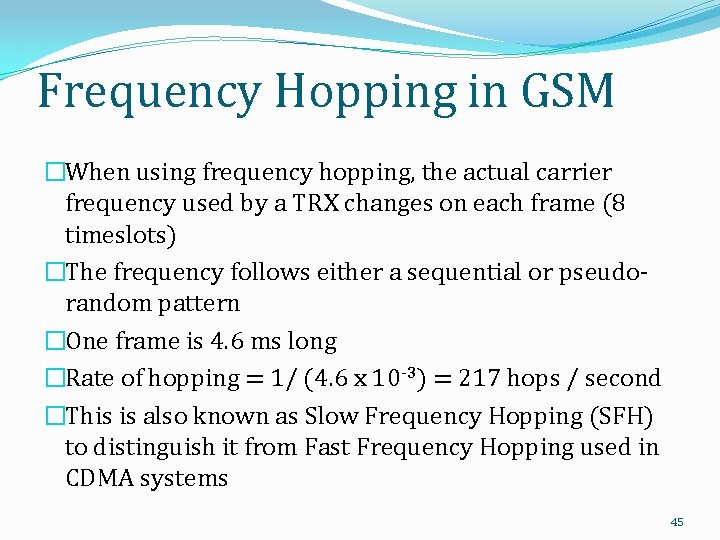
Frequency Hopping in GSM �When using frequency hopping, the actual carrier frequency used by a TRX changes on each frame (8 timeslots) �The frequency follows either a sequential or pseudorandom pattern �One frame is 4. 6 ms long �Rate of hopping = 1/ (4. 6 x 10 -3) = 217 hops / second �This is also known as Slow Frequency Hopping (SFH) to distinguish it from Fast Frequency Hopping used in CDMA systems 45
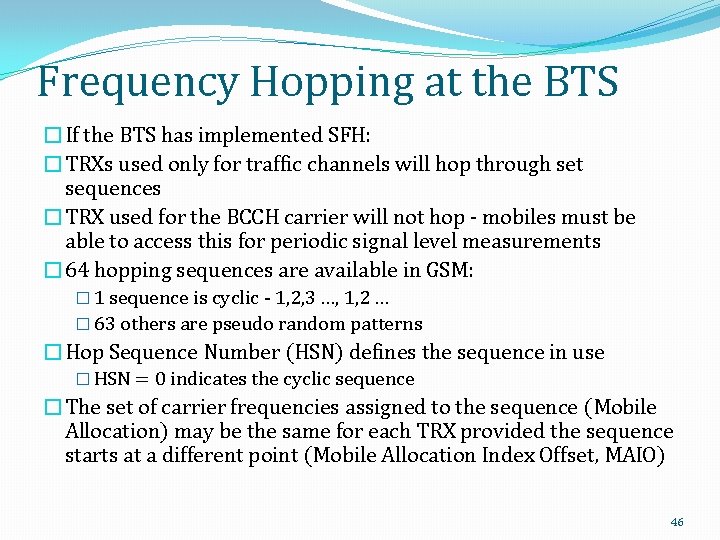
Frequency Hopping at the BTS �If the BTS has implemented SFH: �TRXs used only for traffic channels will hop through set sequences �TRX used for the BCCH carrier will not hop - mobiles must be able to access this for periodic signal level measurements � 64 hopping sequences are available in GSM: � 1 sequence is cyclic - 1, 2, 3 …, 1, 2 … � 63 others are pseudo random patterns �Hop Sequence Number (HSN) defines the sequence in use � HSN = 0 indicates the cyclic sequence �The set of carrier frequencies assigned to the sequence (Mobile Allocation) may be the same for each TRX provided the sequence starts at a different point (Mobile Allocation Index Offset, MAIO) 46
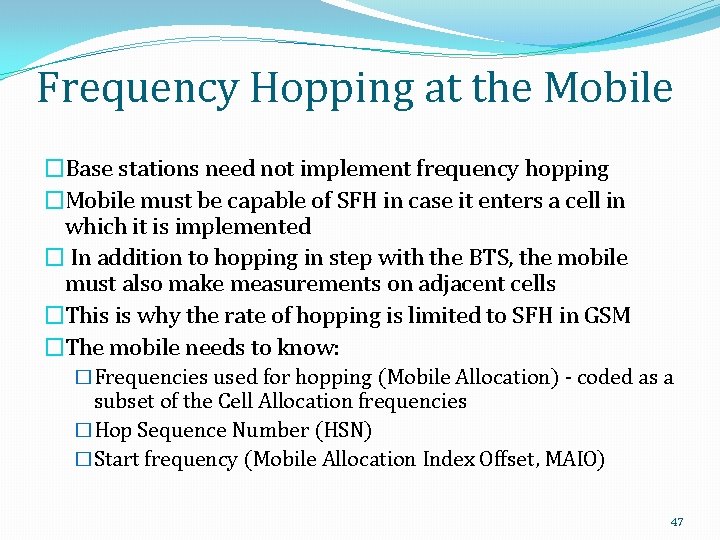
Frequency Hopping at the Mobile �Base stations need not implement frequency hopping �Mobile must be capable of SFH in case it enters a cell in which it is implemented � In addition to hopping in step with the BTS, the mobile must also make measurements on adjacent cells �This is why the rate of hopping is limited to SFH in GSM �The mobile needs to know: �Frequencies used for hopping (Mobile Allocation) - coded as a subset of the Cell Allocation frequencies �Hop Sequence Number (HSN) �Start frequency (Mobile Allocation Index Offset, MAIO) 47
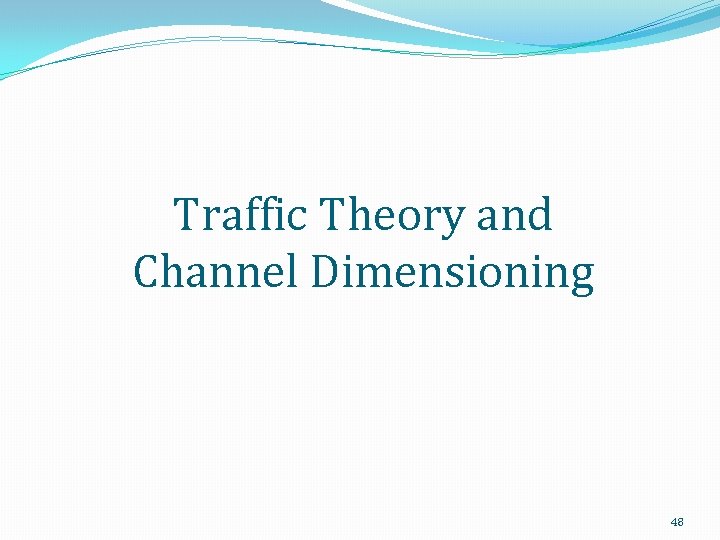
Traffic Theory and Channel Dimensioning 48
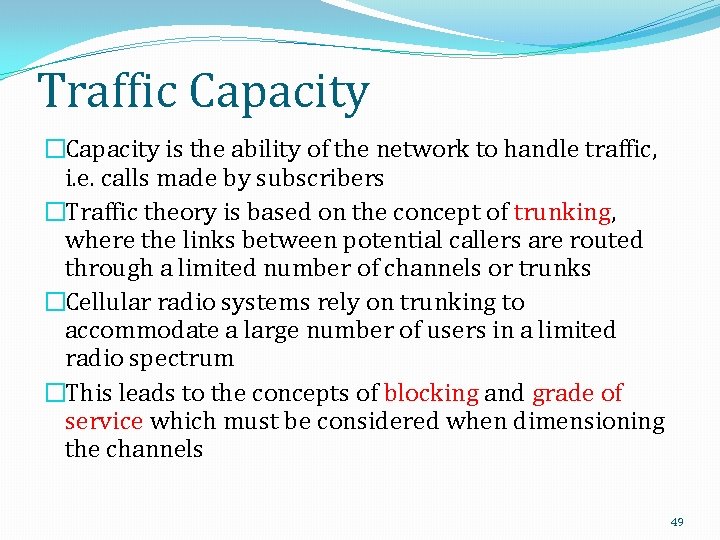
Traffic Capacity �Capacity is the ability of the network to handle traffic, i. e. calls made by subscribers �Traffic theory is based on the concept of trunking, where the links between potential callers are routed through a limited number of channels or trunks �Cellular radio systems rely on trunking to accommodate a large number of users in a limited radio spectrum �This leads to the concepts of blocking and grade of service which must be considered when dimensioning the channels 49
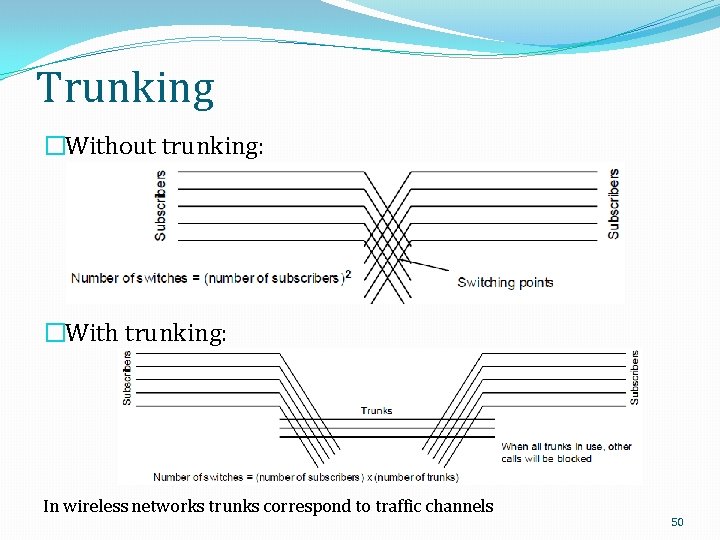
Trunking �Without trunking: �With trunking: In wireless networks trunks correspond to traffic channels 50
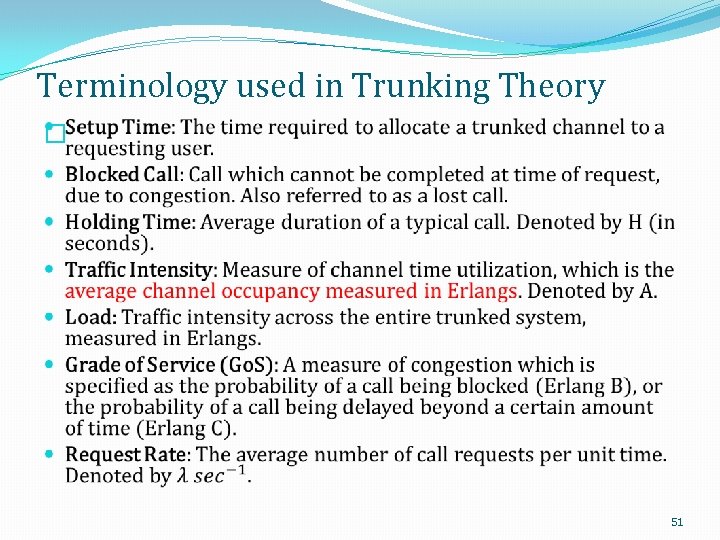
Terminology used in Trunking Theory � 51
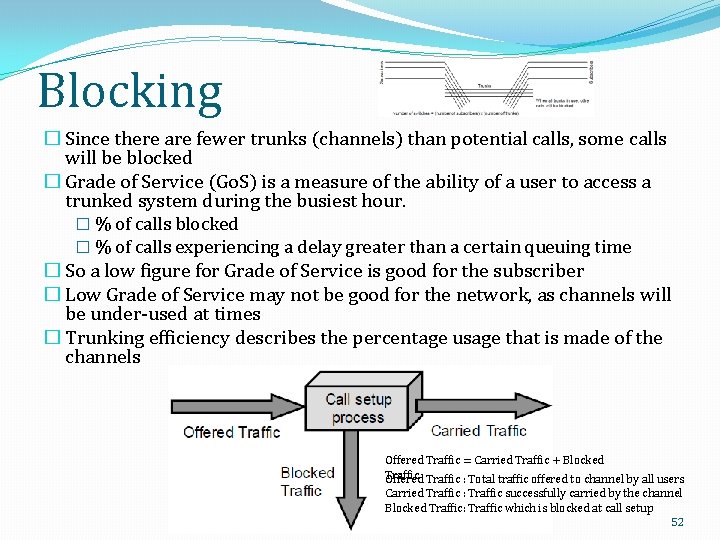
Blocking � Since there are fewer trunks (channels) than potential calls, some calls will be blocked � Grade of Service (Go. S) is a measure of the ability of a user to access a trunked system during the busiest hour. � % of calls blocked � % of calls experiencing a delay greater than a certain queuing time � So a low figure for Grade of Service is good for the subscriber � Low Grade of Service may not be good for the network, as channels will be under-used at times � Trunking efficiency describes the percentage usage that is made of the channels Offered Traffic = Carried Traffic + Blocked Traffic Offered Traffic : Total traffic offered to channel by all users Carried Traffic : Traffic successfully carried by the channel Blocked Traffic: Traffic which is blocked at call setup 52
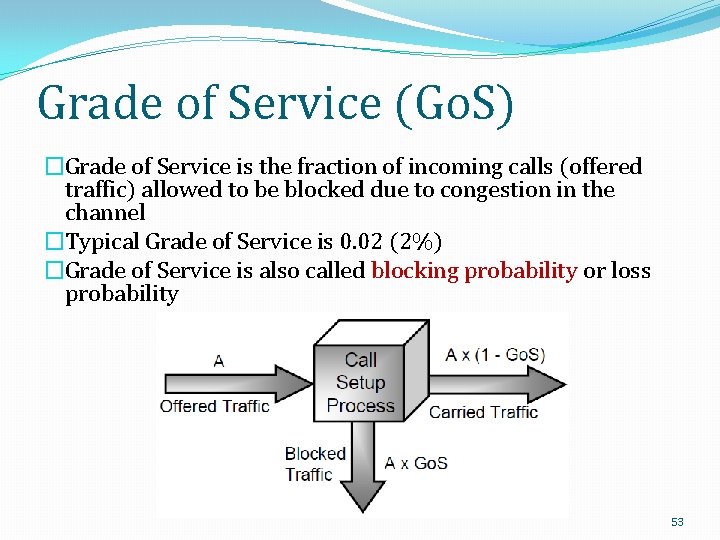
Grade of Service (Go. S) �Grade of Service is the fraction of incoming calls (offered traffic) allowed to be blocked due to congestion in the channel �Typical Grade of Service is 0. 02 (2%) �Grade of Service is also called blocking probability or loss probability 53
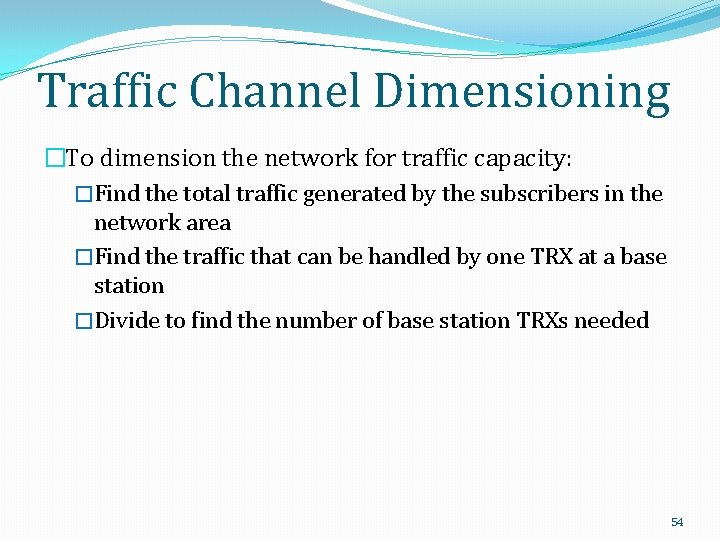
Traffic Channel Dimensioning �To dimension the network for traffic capacity: �Find the total traffic generated by the subscribers in the network area �Find the traffic that can be handled by one TRX at a base station �Divide to find the number of base station TRXs needed 54
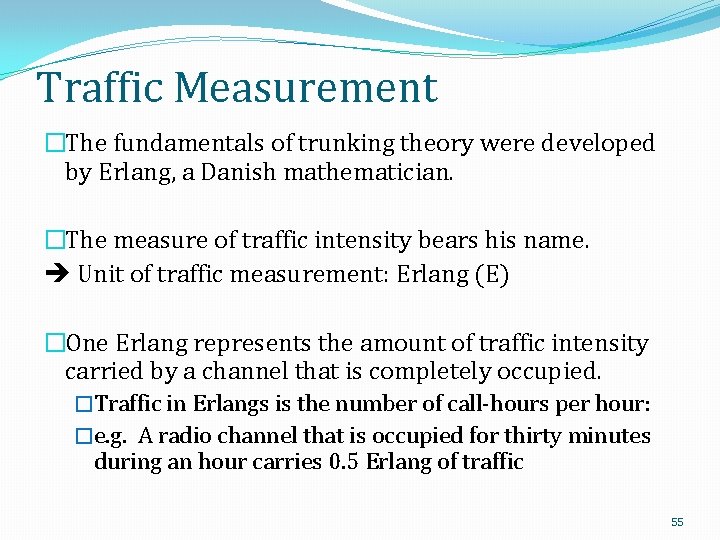
Traffic Measurement �The fundamentals of trunking theory were developed by Erlang, a Danish mathematician. �The measure of traffic intensity bears his name. Unit of traffic measurement: Erlang (E) �One Erlang represents the amount of traffic intensity carried by a channel that is completely occupied. �Traffic in Erlangs is the number of call-hours per hour: �e. g. A radio channel that is occupied for thirty minutes during an hour carries 0. 5 Erlang of traffic 55
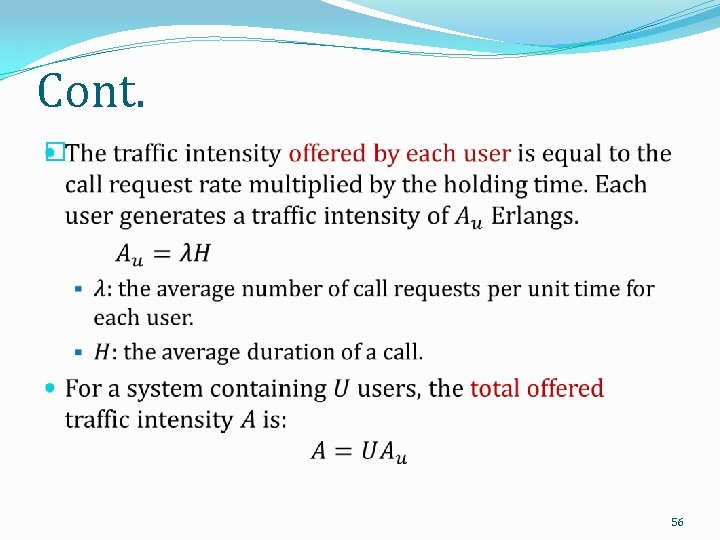
Cont. � 56
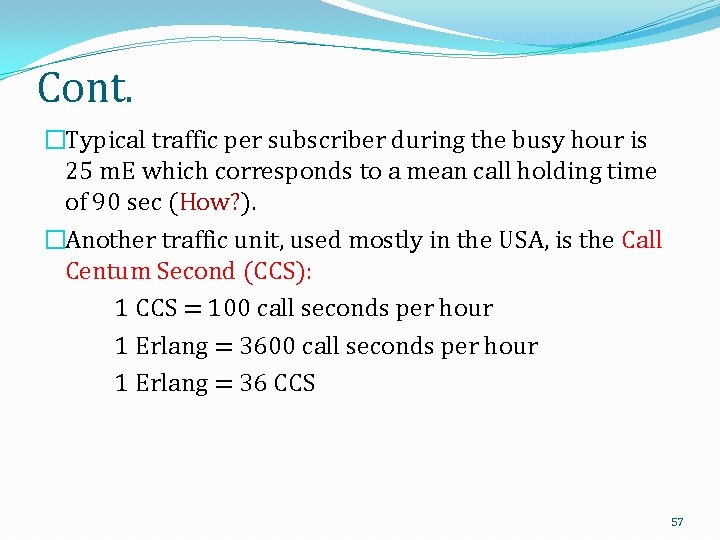
Cont. �Typical traffic per subscriber during the busy hour is 25 m. E which corresponds to a mean call holding time of 90 sec (How? ). �Another traffic unit, used mostly in the USA, is the Call Centum Second (CCS): 1 CCS = 100 call seconds per hour 1 Erlang = 36 CCS 57
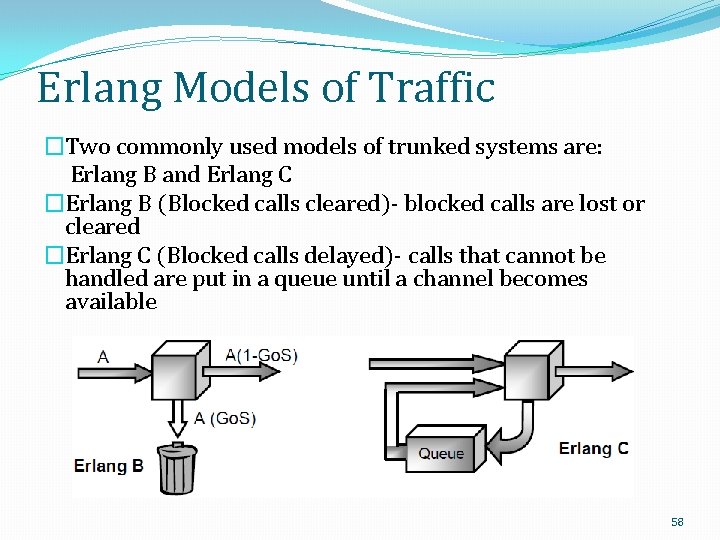
Erlang Models of Traffic �Two commonly used models of trunked systems are: Erlang B and Erlang C �Erlang B (Blocked calls cleared)- blocked calls are lost or cleared �Erlang C (Blocked calls delayed)- calls that cannot be handled are put in a queue until a channel becomes available 58
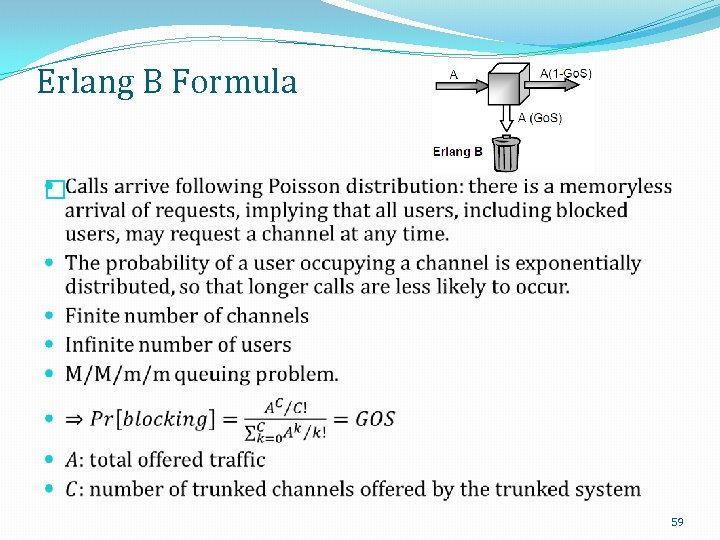
Erlang B Formula � 59
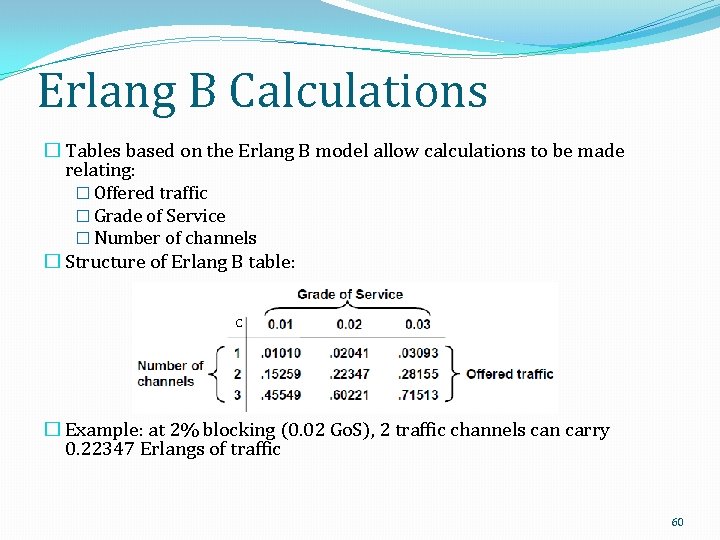
Erlang B Calculations � Tables based on the Erlang B model allow calculations to be made relating: � Offered traffic � Grade of Service � Number of channels � Structure of Erlang B table: C � Example: at 2% blocking (0. 02 Go. S), 2 traffic channels can carry 0. 22347 Erlangs of traffic 60
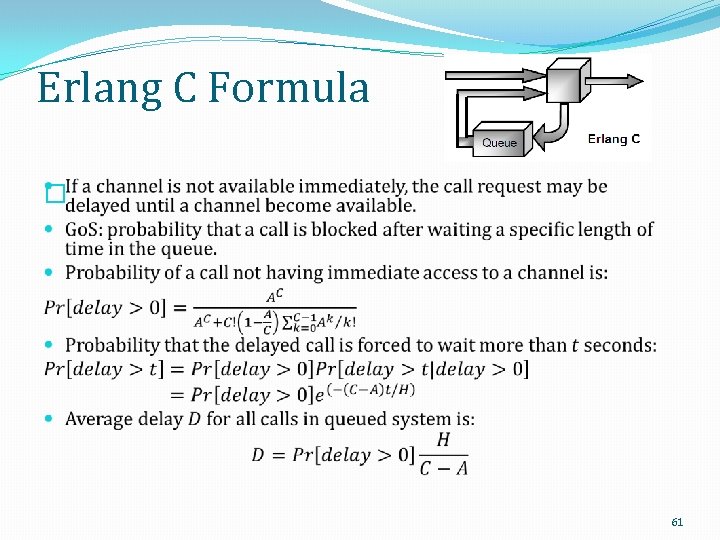
Erlang C Formula � 61
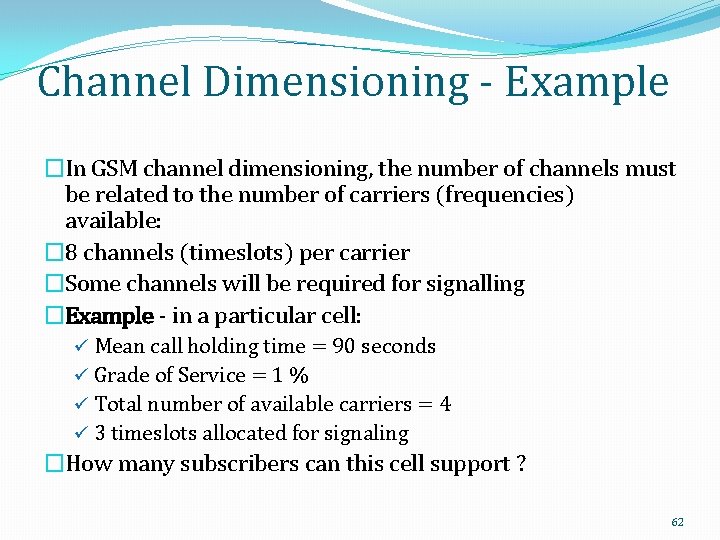
Channel Dimensioning - Example �In GSM channel dimensioning, the number of channels must be related to the number of carriers (frequencies) available: � 8 channels (timeslots) per carrier �Some channels will be required for signalling �Example - in a particular cell: ü Mean call holding time = 90 seconds ü Grade of Service = 1 % ü Total number of available carriers = 4 ü 3 timeslots allocated for signaling �How many subscribers can this cell support ? 62
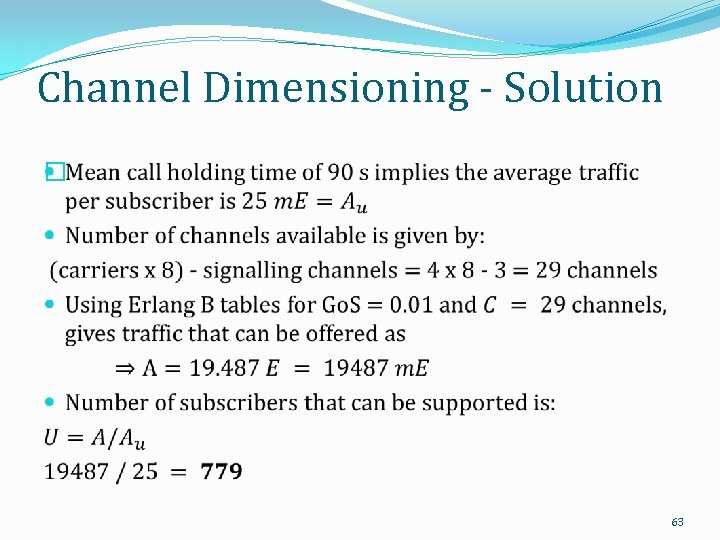
Channel Dimensioning - Solution � 63
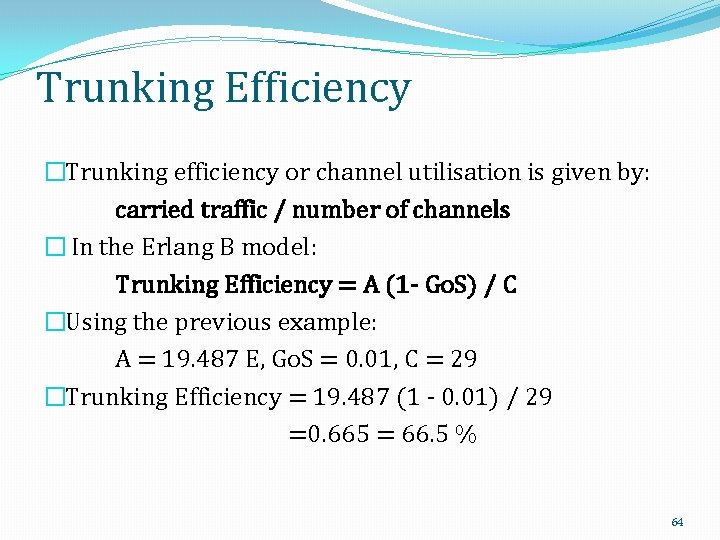
Trunking Efficiency �Trunking efficiency or channel utilisation is given by: carried traffic / number of channels � In the Erlang B model: Trunking Efficiency = A (1 - Go. S) / C �Using the previous example: A = 19. 487 E, Go. S = 0. 01, C = 29 �Trunking Efficiency = 19. 487 (1 - 0. 01) / 29 =0. 665 = 66. 5 % 64
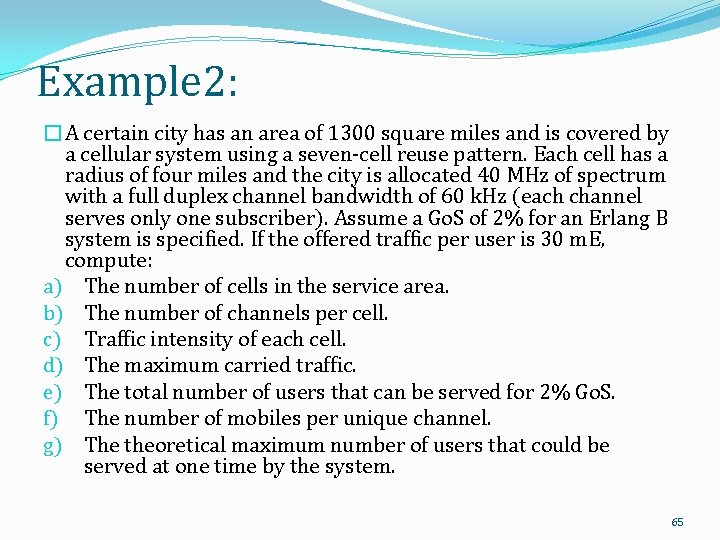
Example 2: �A certain city has an area of 1300 square miles and is covered by a cellular system using a seven-cell reuse pattern. Each cell has a radius of four miles and the city is allocated 40 MHz of spectrum with a full duplex channel bandwidth of 60 k. Hz (each channel serves only one subscriber). Assume a Go. S of 2% for an Erlang B system is specified. If the offered traffic per user is 30 m. E, compute: a) The number of cells in the service area. b) The number of channels per cell. c) Traffic intensity of each cell. d) The maximum carried traffic. e) The total number of users that can be served for 2% Go. S. f) The number of mobiles per unique channel. g) The theoretical maximum number of users that could be served at one time by the system. 65
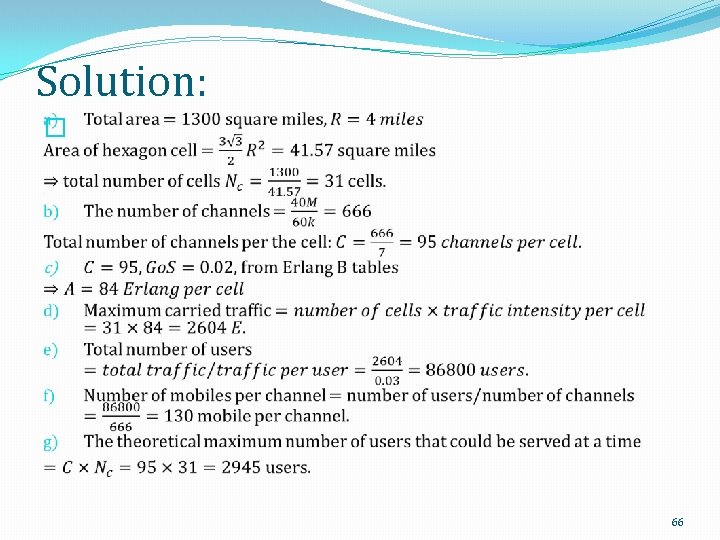
Solution: � 66
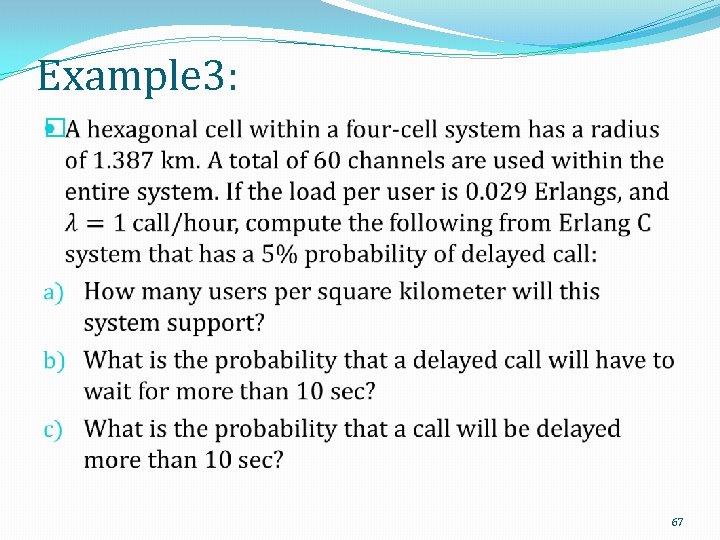
Example 3: � 67
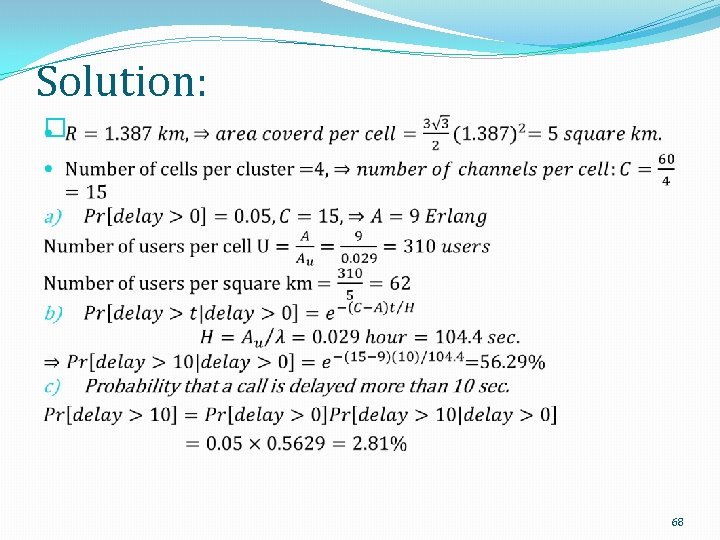
Solution: � 68
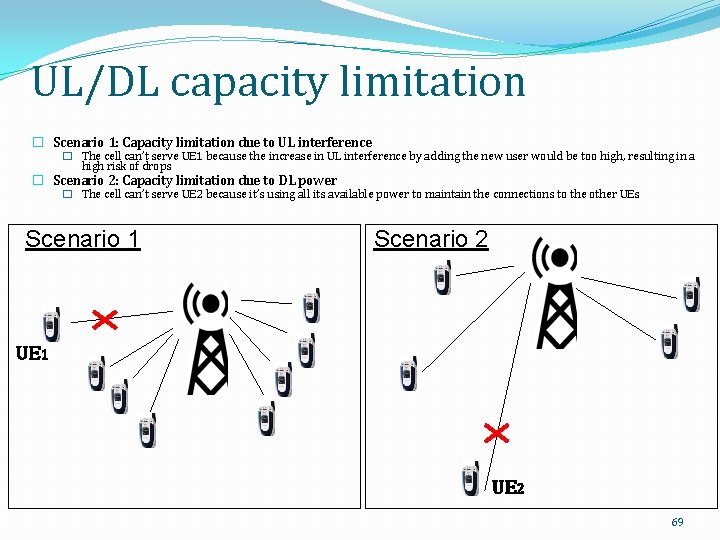
UL/DL capacity limitation � Scenario 1: Capacity limitation due to UL interference � The cell can’t serve UE 1 because the increase in UL interference by adding the new user would be too high, resulting in a high risk of drops � Scenario 2: Capacity limitation due to DL power � The cell can’t serve UE 2 because it’s using all its available power to maintain the connections to the other UEs Scenario 1 Scenario 2 UE 1 UE 2 69
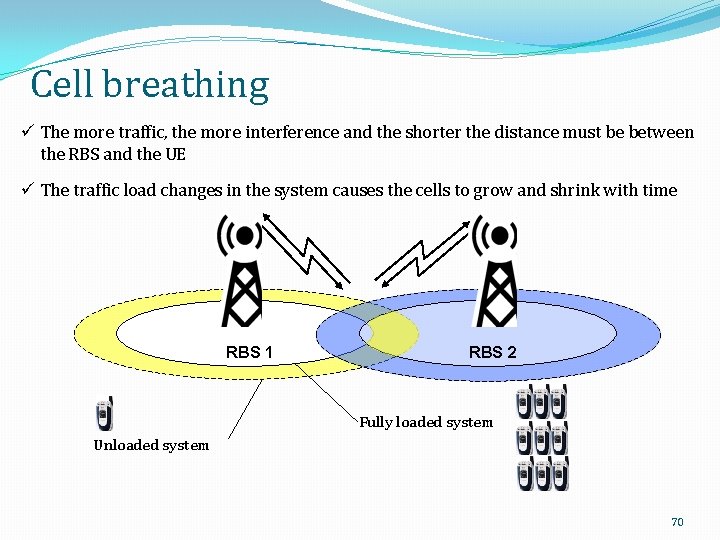
Cell breathing ü The more traffic, the more interference and the shorter the distance must be between the RBS and the UE ü The traffic load changes in the system causes the cells to grow and shrink with time RBS 1 RBS 2 Fully loaded system Unloaded system 70
Radio capacity may be increased in cellular concept by *
Duty to accommodate definition
Preoperational stage
Define accommodate
Drc uic
Duty to accommodate alberta
Conventional radio system
Concept 5 cellular respiration
Concept map cellular respiration
Operation of cellular systems
Hát kết hợp bộ gõ cơ thể
Frameset trong html5
Bổ thể
Tỉ lệ cơ thể trẻ em
Chó sói
Tư thế worms-breton
Bài hát chúa yêu trần thế alleluia
Môn thể thao bắt đầu bằng từ đua
Thế nào là hệ số cao nhất
Các châu lục và đại dương trên thế giới
Công thức tính thế năng
Trời xanh đây là của chúng ta thể thơ
Mật thư anh em như thể tay chân
101012 bằng
độ dài liên kết
Các châu lục và đại dương trên thế giới
Thể thơ truyền thống
Quá trình desamine hóa có thể tạo ra
Một số thể thơ truyền thống
Cái miệng nó xinh thế
Vẽ hình chiếu vuông góc của vật thể sau
Biện pháp chống mỏi cơ
đặc điểm cơ thể của người tối cổ
V cc cc
Vẽ hình chiếu đứng bằng cạnh của vật thể
Phối cảnh
Thẻ vin
đại từ thay thế
điện thế nghỉ
Tư thế ngồi viết
Diễn thế sinh thái là
Các loại đột biến cấu trúc nhiễm sắc thể
Bảng số nguyên tố
Tư thế ngồi viết
Lời thề hippocrates
Thiếu nhi thế giới liên hoan
ưu thế lai là gì
Khi nào hổ mẹ dạy hổ con săn mồi
Sự nuôi và dạy con của hươu
Sơ đồ cơ thể người
Từ ngữ thể hiện lòng nhân hậu
Thế nào là mạng điện lắp đặt kiểu nổi
Examples of ideal self
Pengertian marketing concept
Concept map neuman systems model
Decision support systems and intelligent systems
Principles of complex systems for systems engineering
Embedded systems vs cyber physical systems
Engineering elegant systems: theory of systems engineering
Molecular level vs cellular level
Cellular respiration redox
What is the correct equation for cellular respiration?
Chemiosmosis steps
Aerobic vs anaerobic
Lactic acid fermentation equation
Why is cellular respiration important
Porifera body organization
Complimentary processes
What's the equation for cellular respiration
Cellular respiration reactants
Cellular respiration and photosynthesis equation