Molecules in motion Different states of matter different
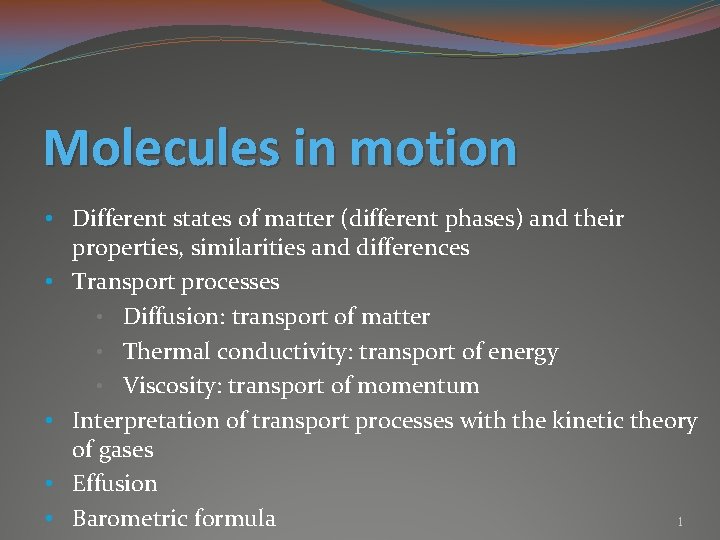
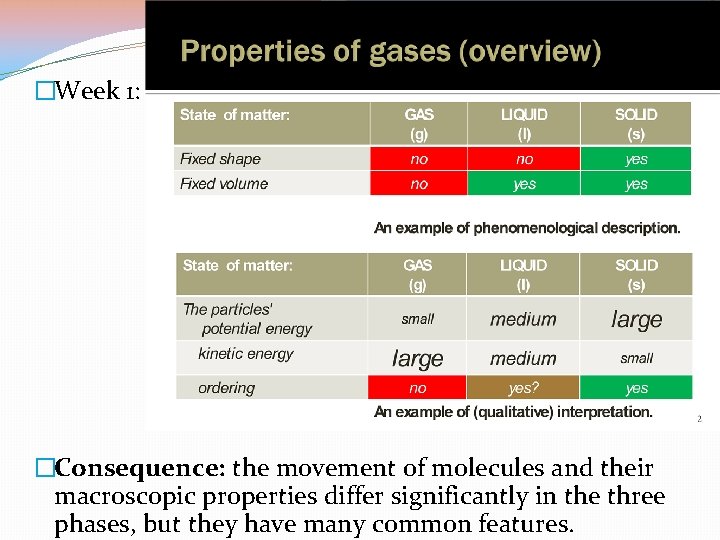
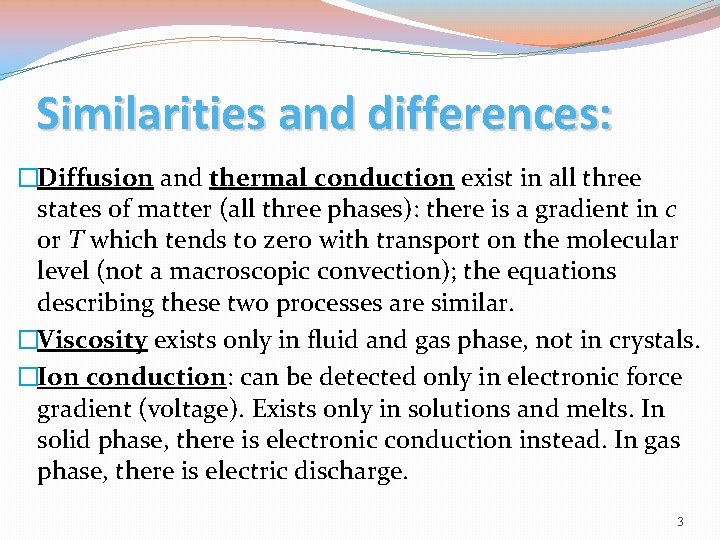
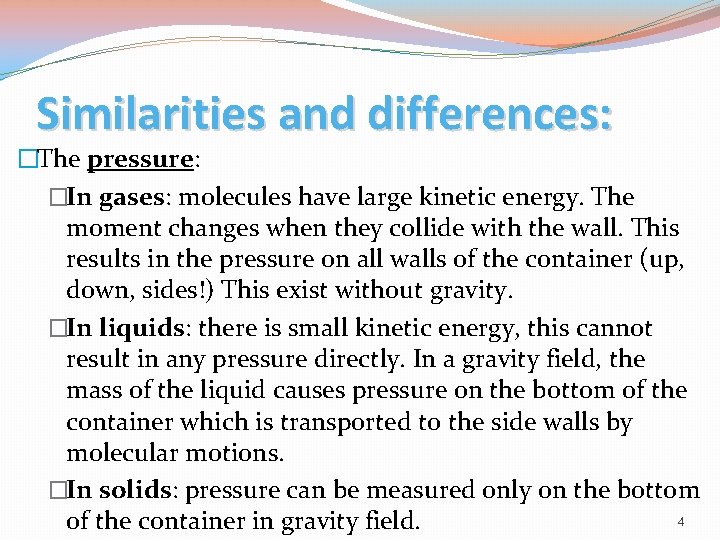
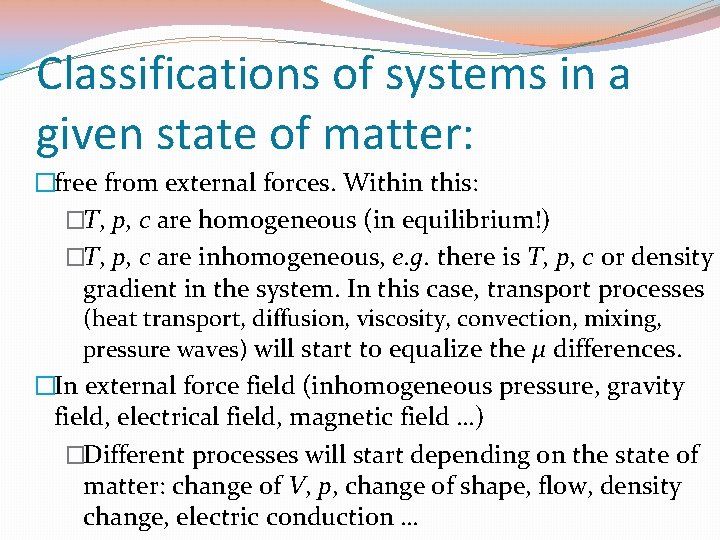
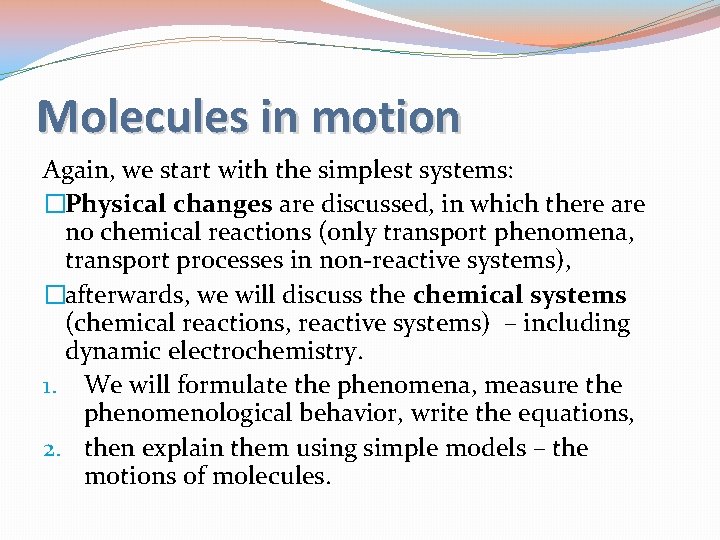
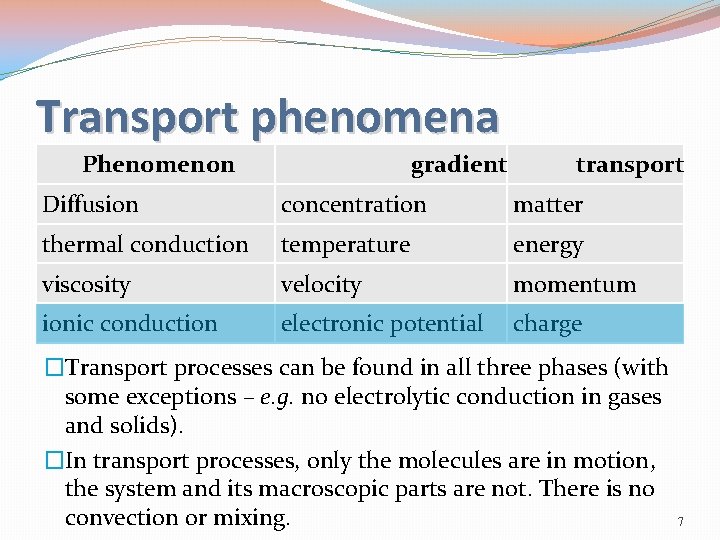
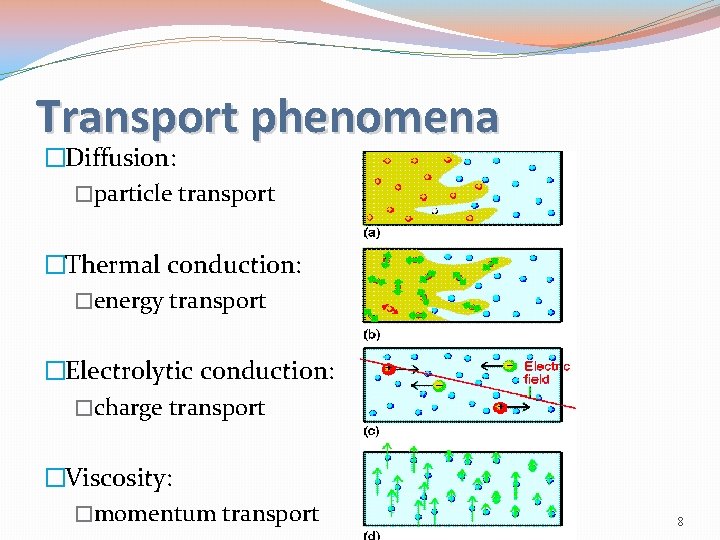
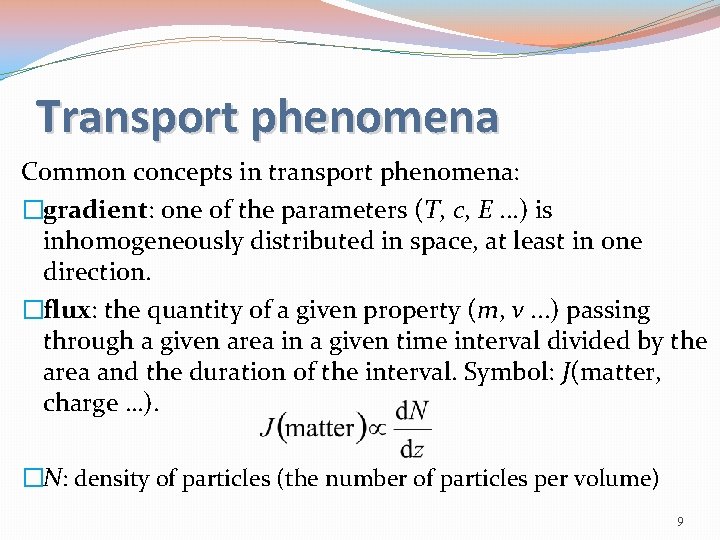
![Diffusion: transport of matter (at molecular level) [J]: m-2 s-1 [D]: m 2 s-1 Diffusion: transport of matter (at molecular level) [J]: m-2 s-1 [D]: m 2 s-1](https://slidetodoc.com/presentation_image_h2/81d4bb159f6fcac9231140af0e61230c/image-10.jpg)
![Thermal conduction: transport of energy [J]: [κ]: J m-2 s-1 flux of energy J Thermal conduction: transport of energy [J]: [κ]: J m-2 s-1 flux of energy J](https://slidetodoc.com/presentation_image_h2/81d4bb159f6fcac9231140af0e61230c/image-11.jpg)
![Viscosity: transport of momentum [J]: [η]: kg m-1 s-2 kg m-1 s-1 flux of Viscosity: transport of momentum [J]: [η]: kg m-1 s-2 kg m-1 s-1 flux of](https://slidetodoc.com/presentation_image_h2/81d4bb159f6fcac9231140af0e61230c/image-12.jpg)
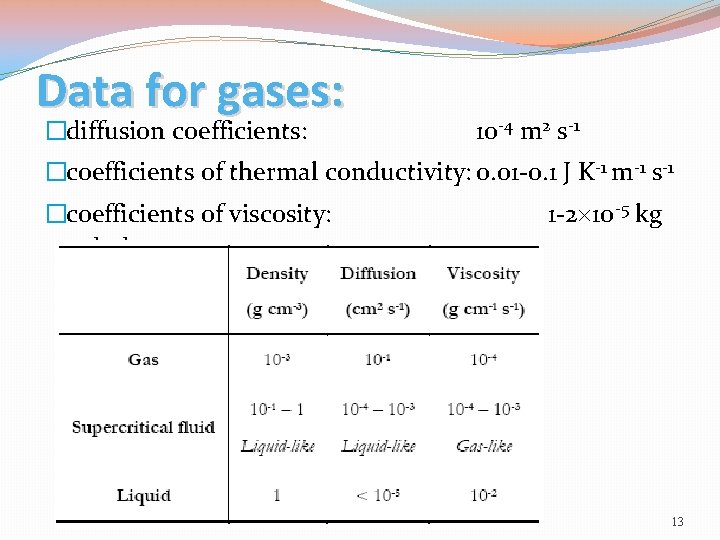
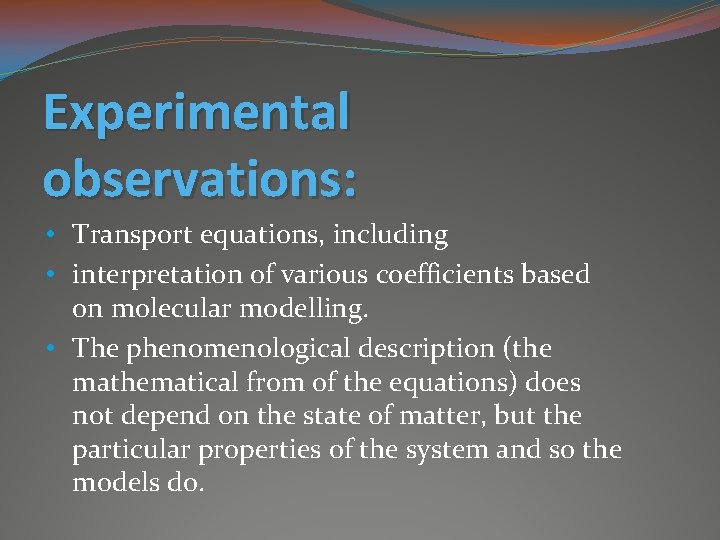
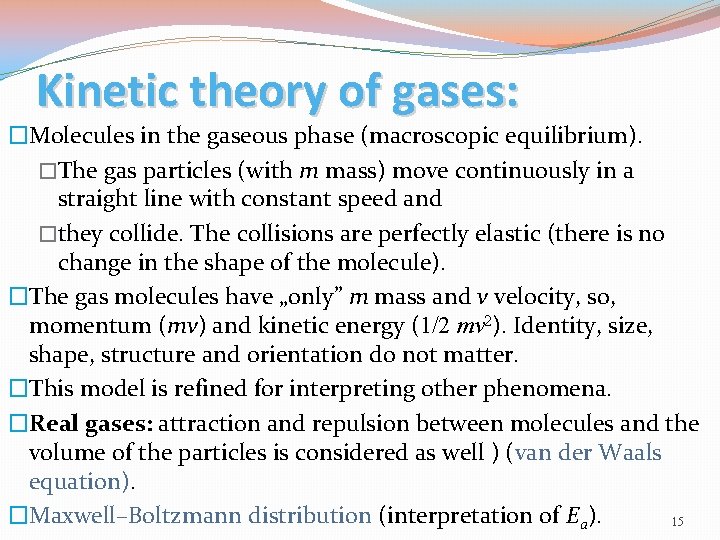
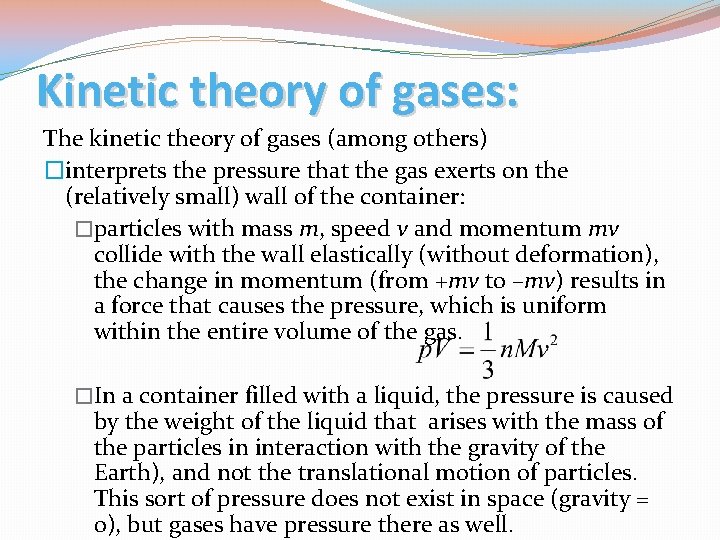
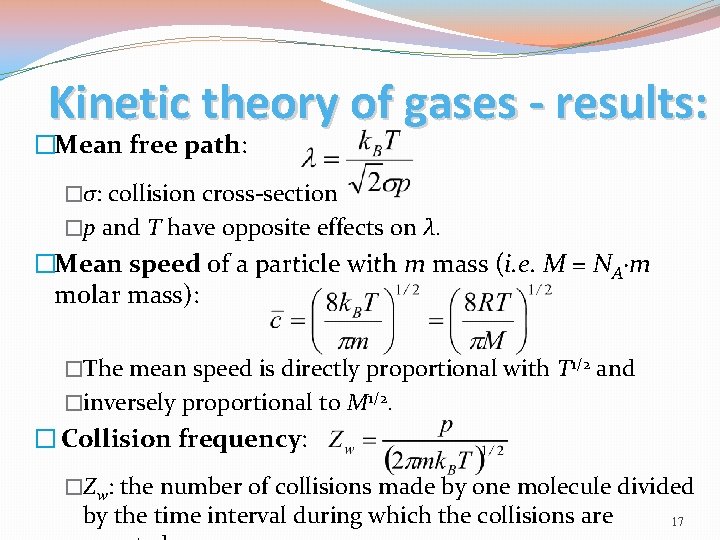
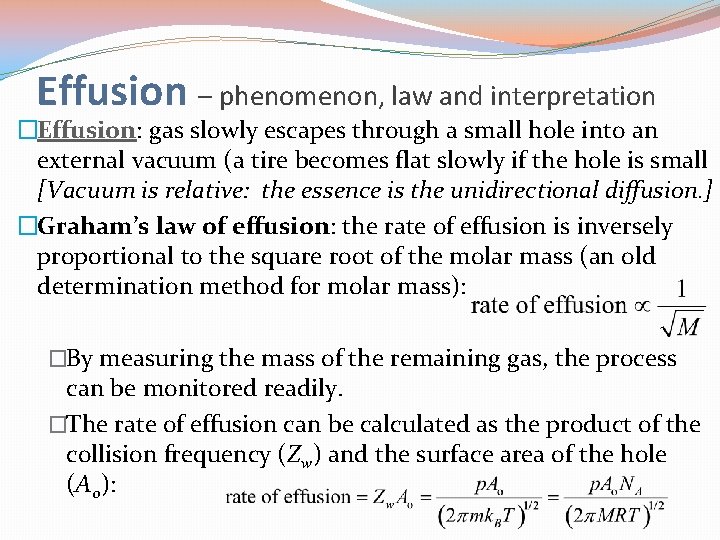
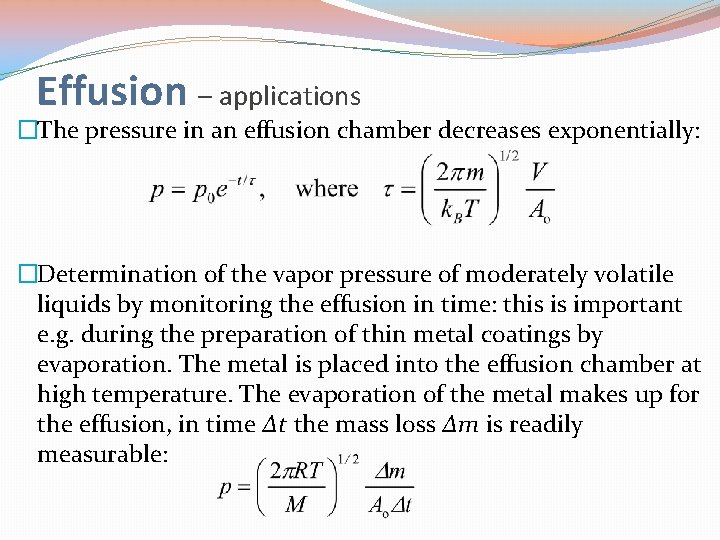
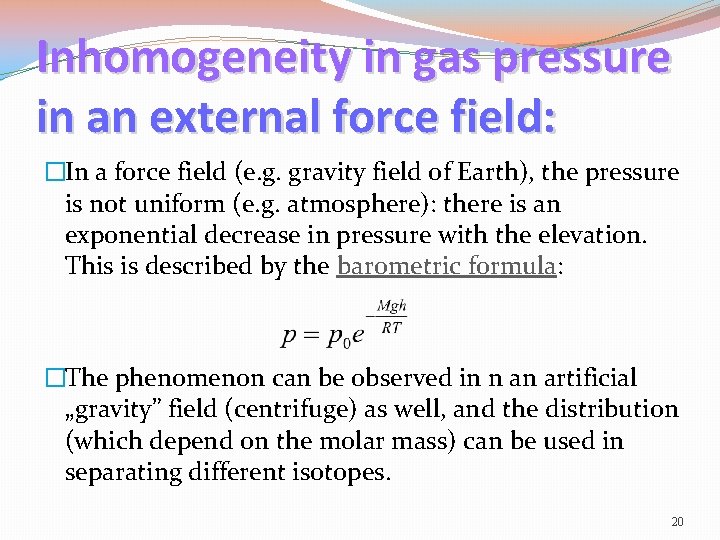
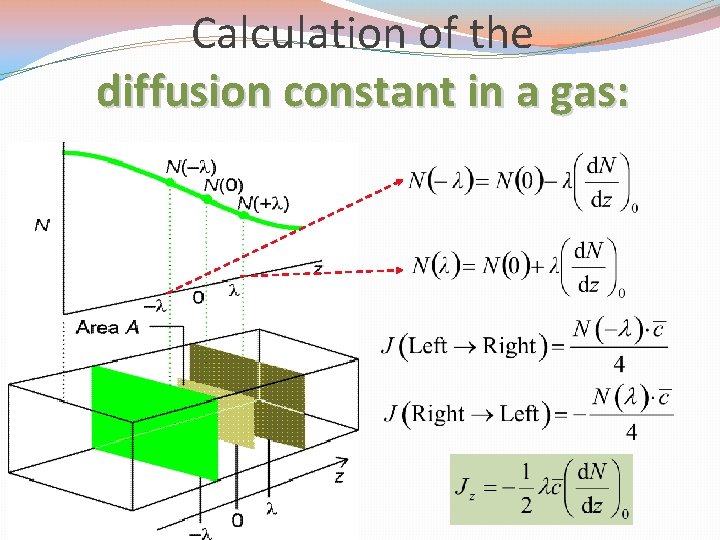
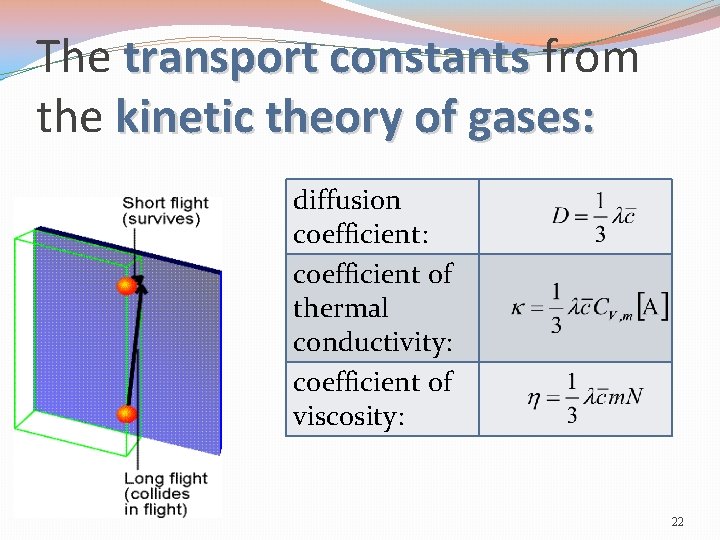
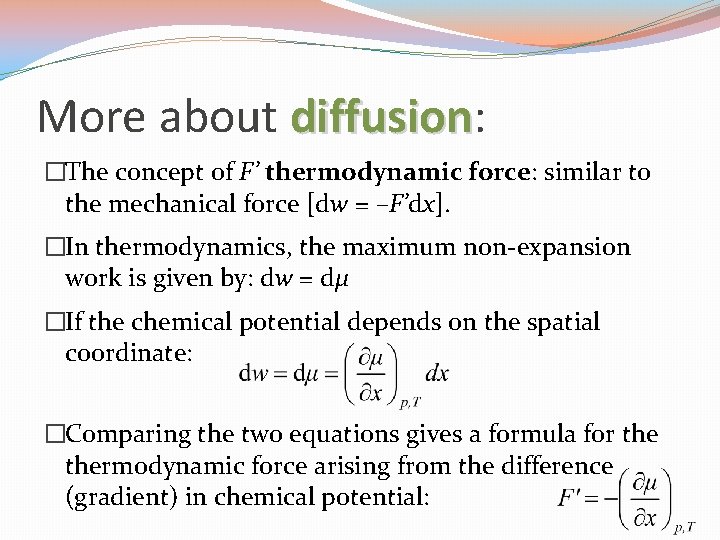
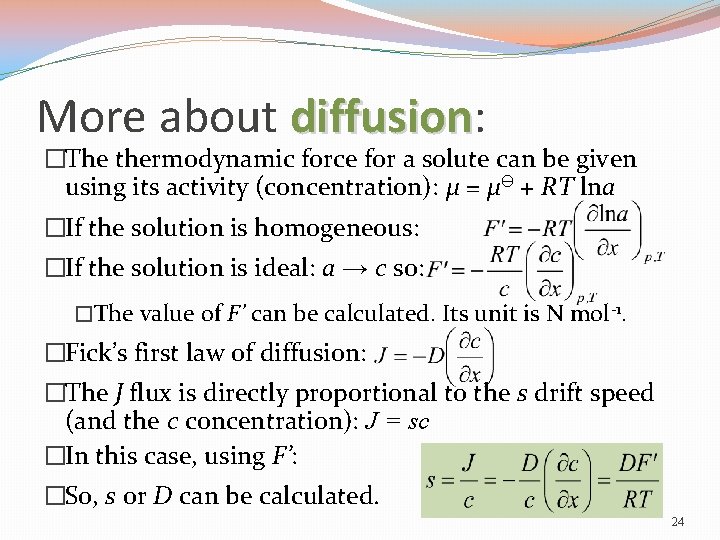
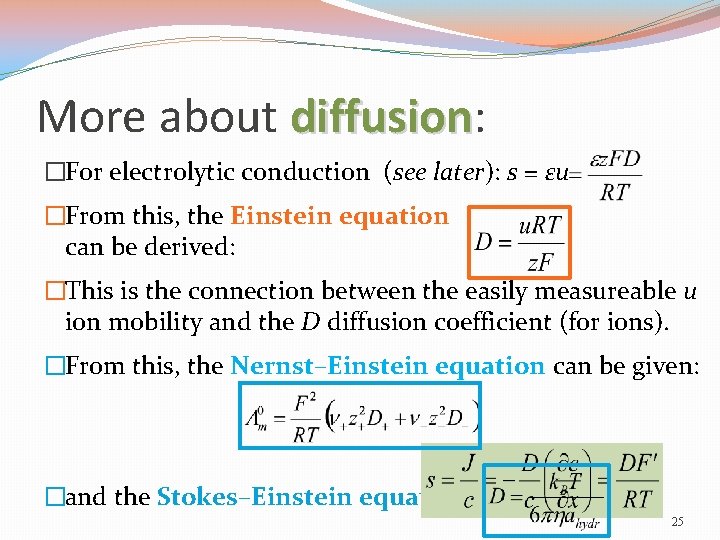
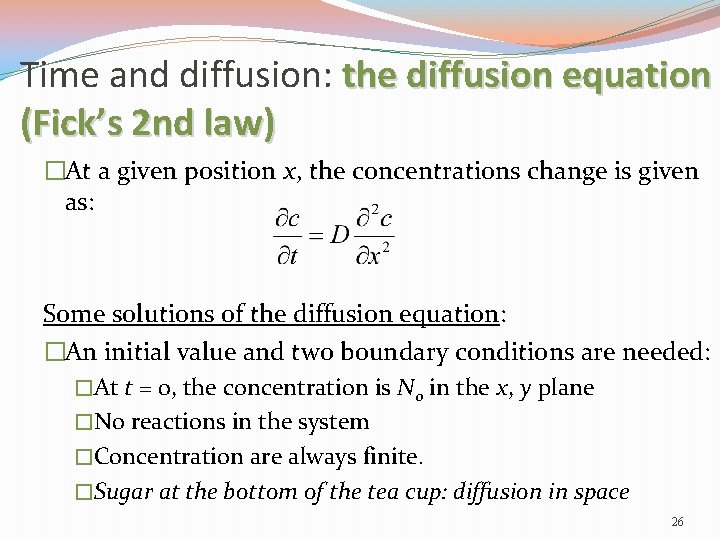
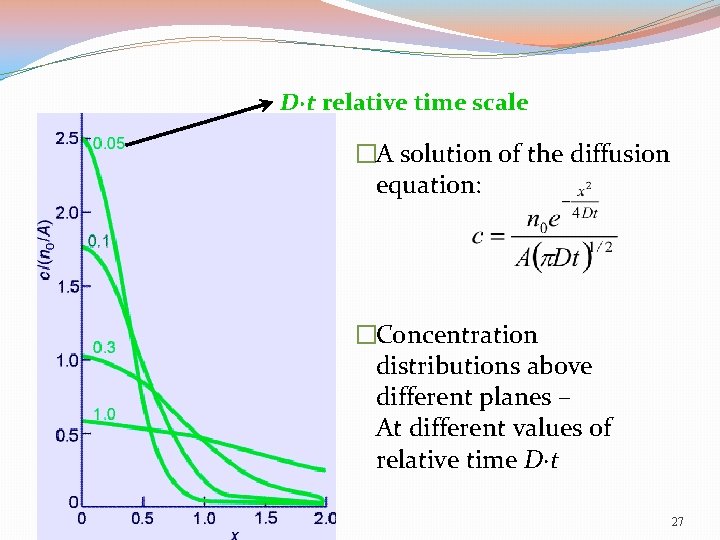
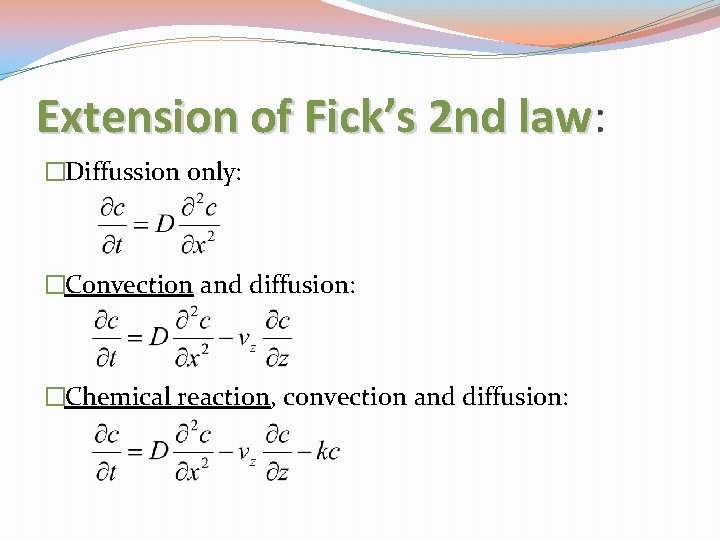
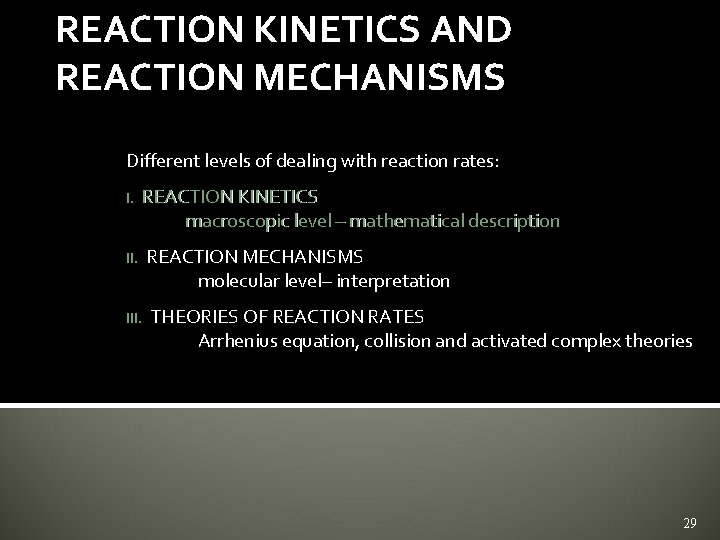
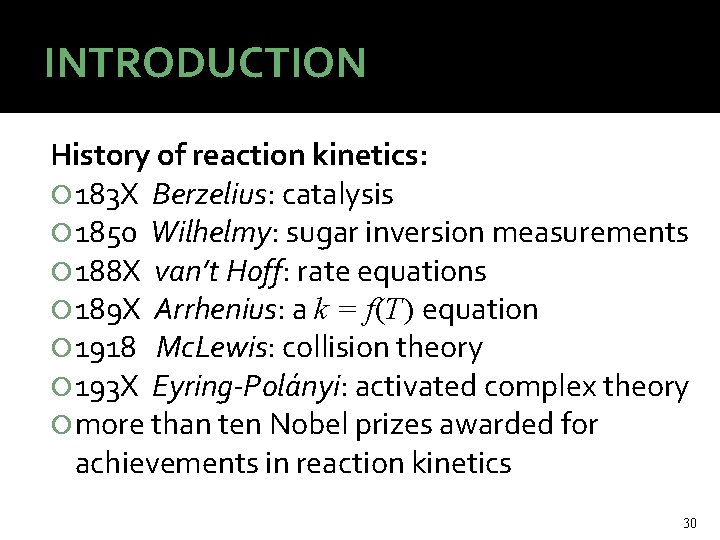
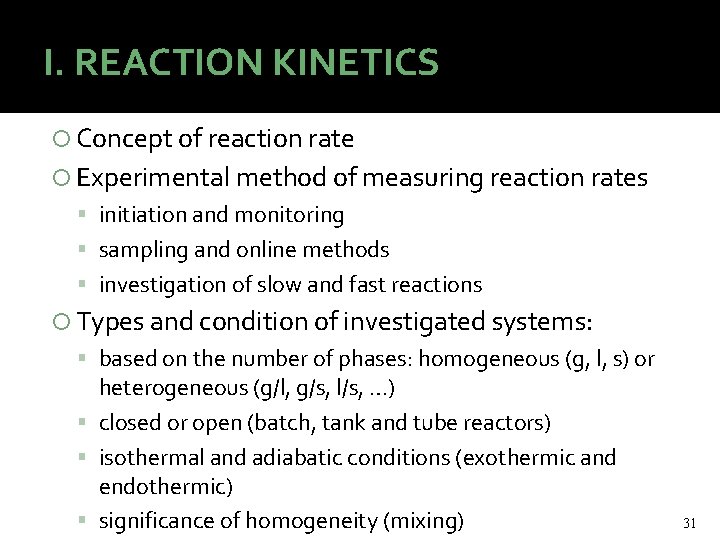
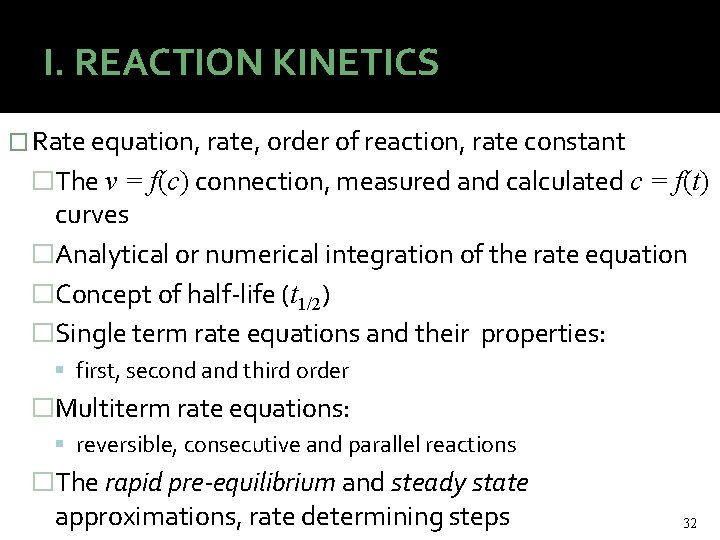
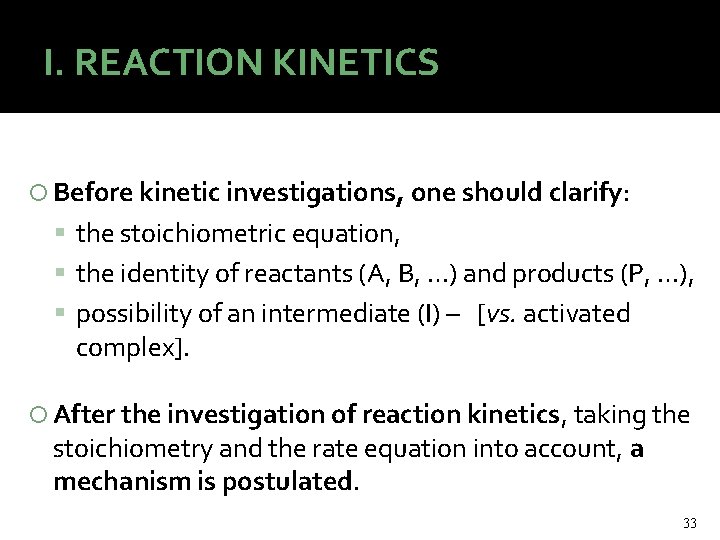
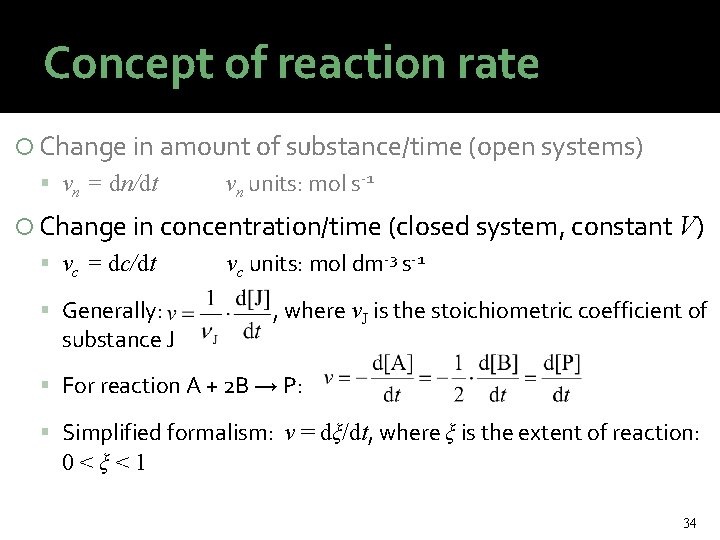
![Visualizing the reaction rate – graphical representation Rate equation: v = dc/dt = k[A]α[B]β[C]γ… Visualizing the reaction rate – graphical representation Rate equation: v = dc/dt = k[A]α[B]β[C]γ…](https://slidetodoc.com/presentation_image_h2/81d4bb159f6fcac9231140af0e61230c/image-35.jpg)
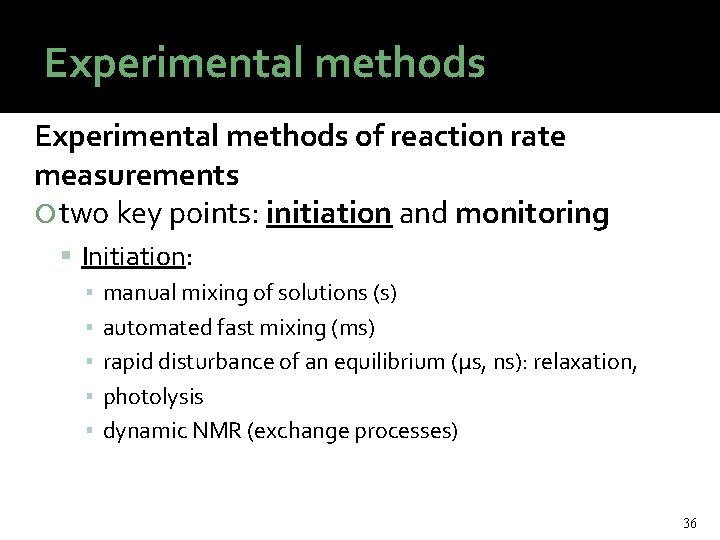
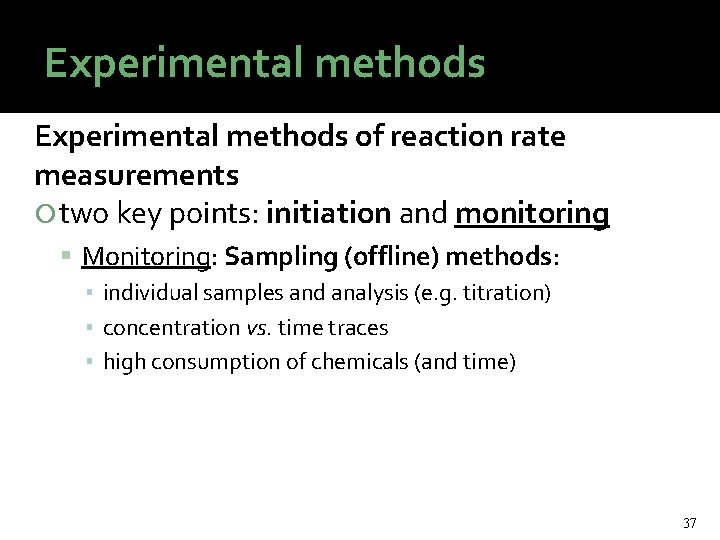
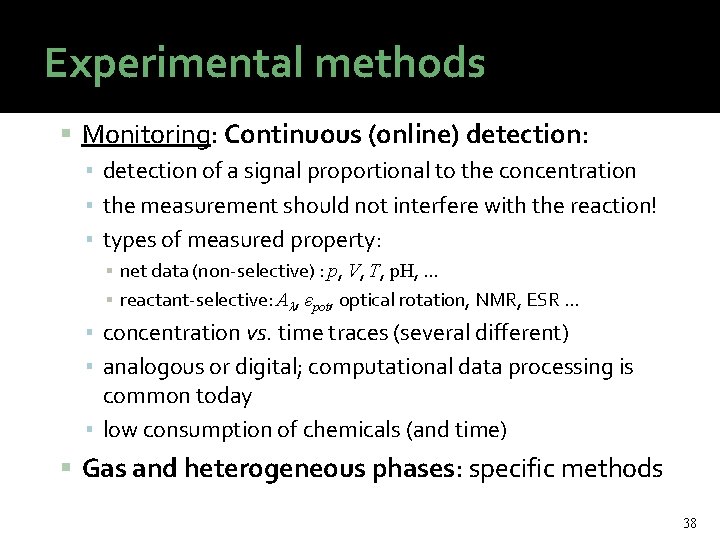
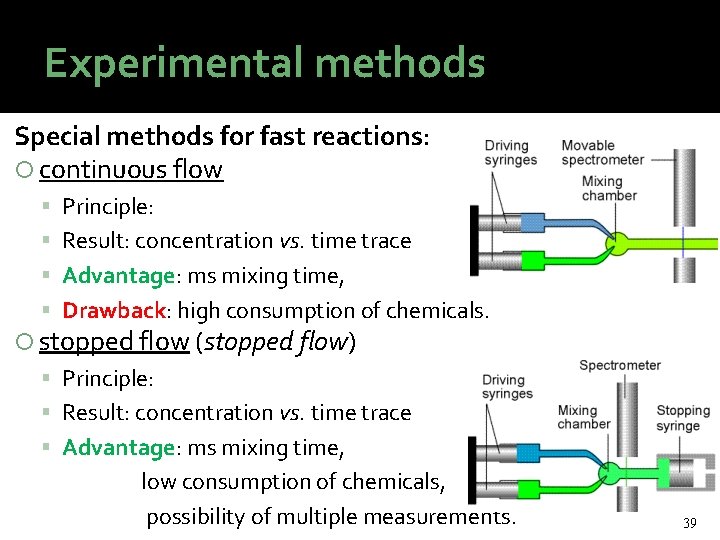
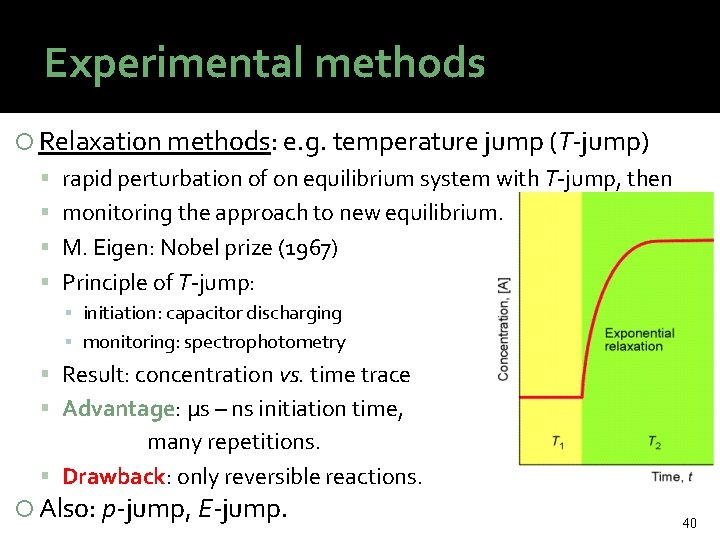
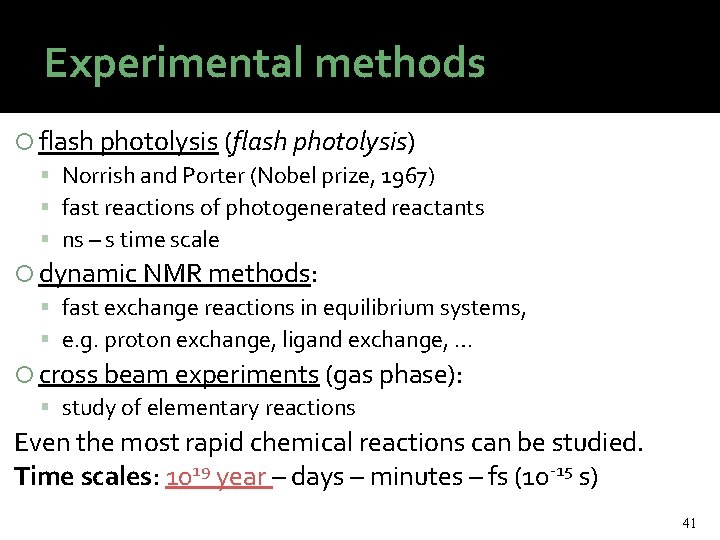
![Rate equation � v = dc/dt = k[A]α[B]β[C]γ… v: rate of reaction k: rate Rate equation � v = dc/dt = k[A]α[B]β[C]γ… v: rate of reaction k: rate](https://slidetodoc.com/presentation_image_h2/81d4bb159f6fcac9231140af0e61230c/image-42.jpg)
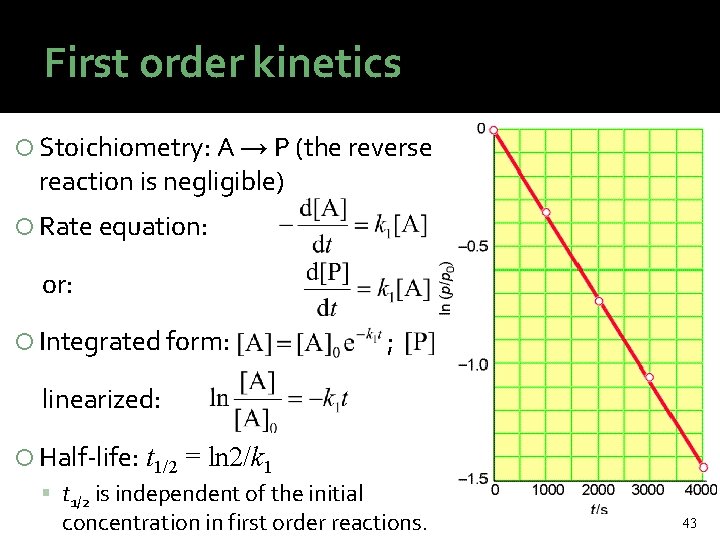
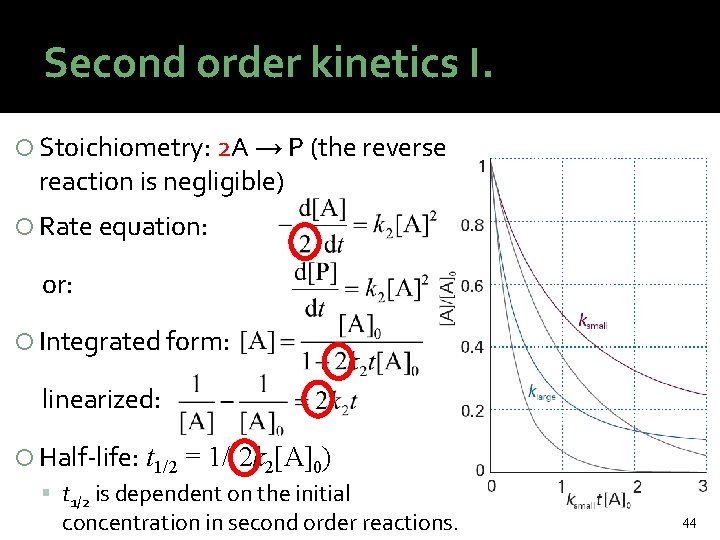
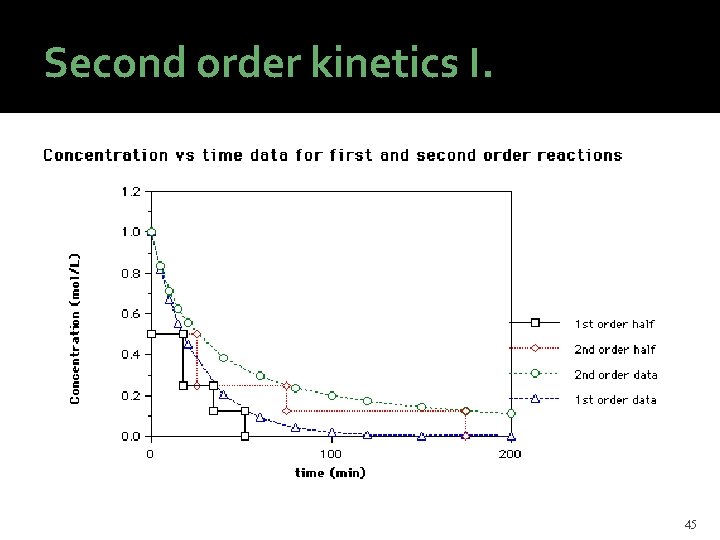
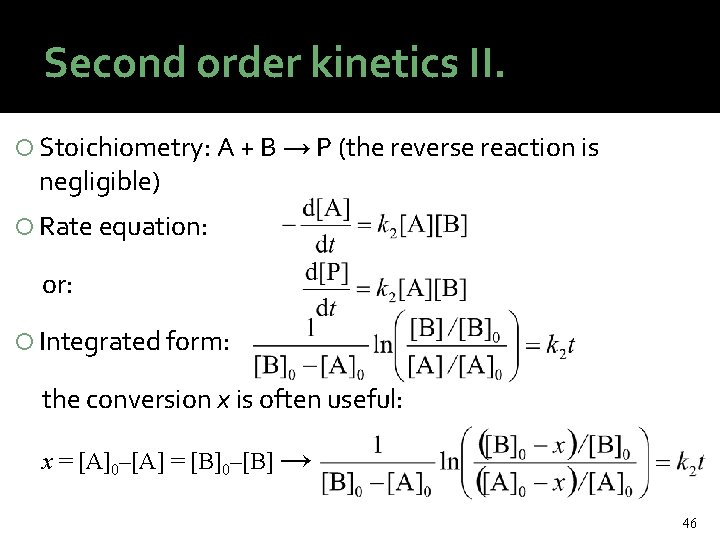
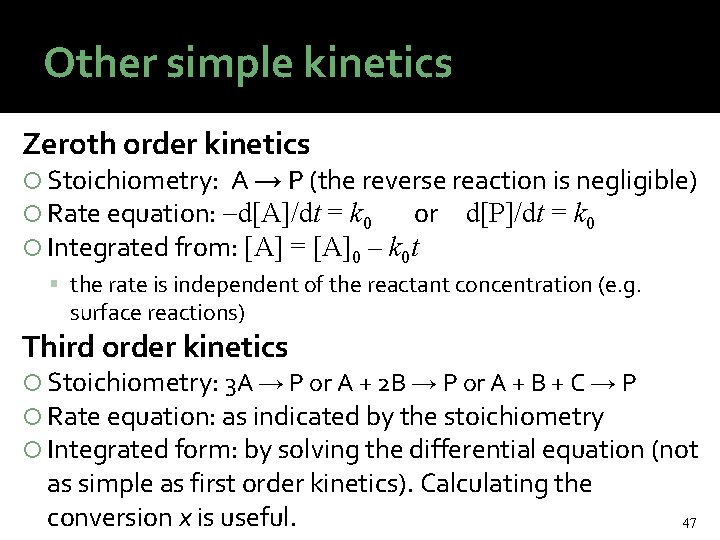
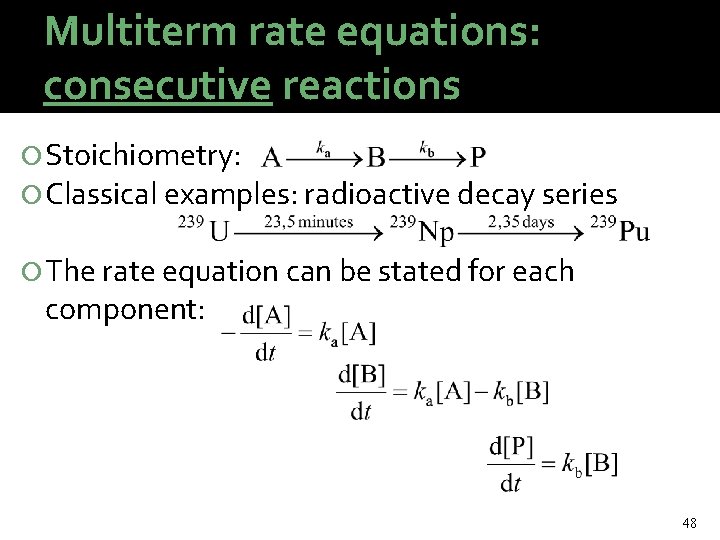
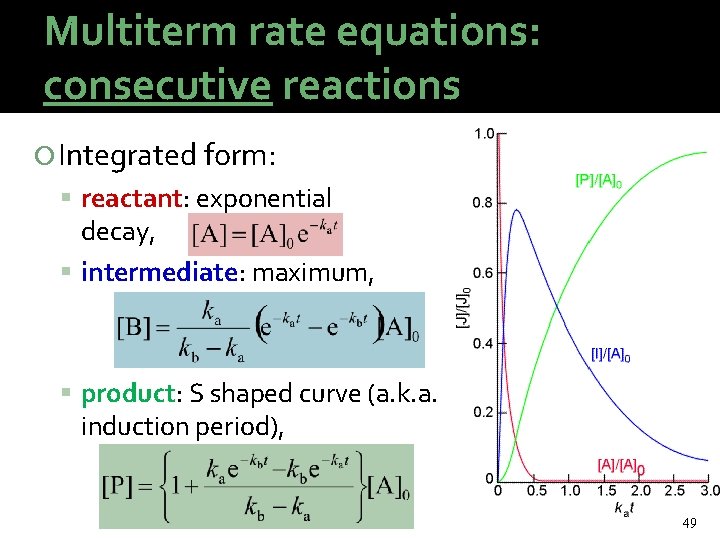
![Multiterm rate equations: parallel reactions Relatively simple (close to obvious) Stoichiometry: d[P 1]/dt = Multiterm rate equations: parallel reactions Relatively simple (close to obvious) Stoichiometry: d[P 1]/dt =](https://slidetodoc.com/presentation_image_h2/81d4bb159f6fcac9231140af0e61230c/image-50.jpg)
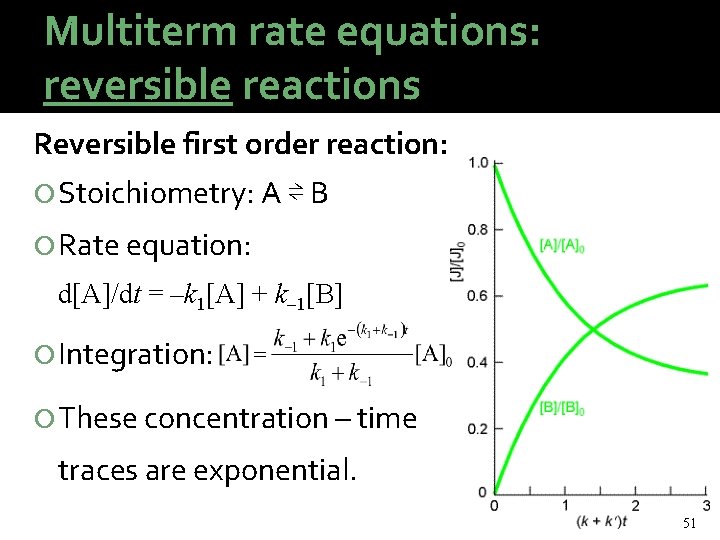
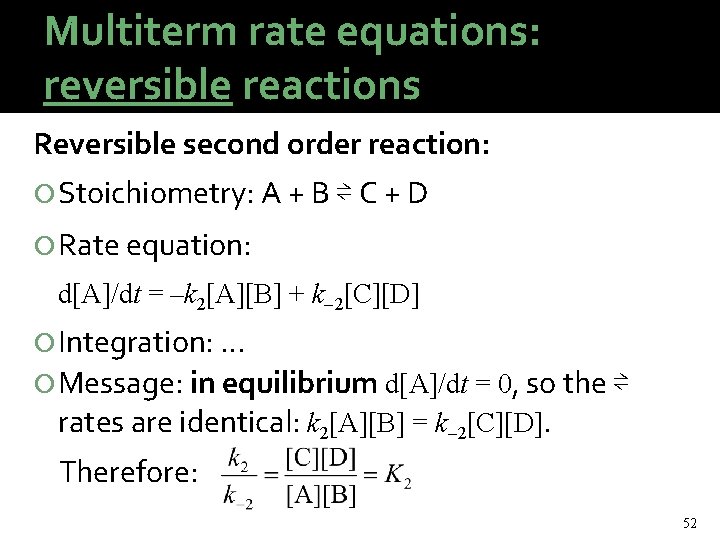
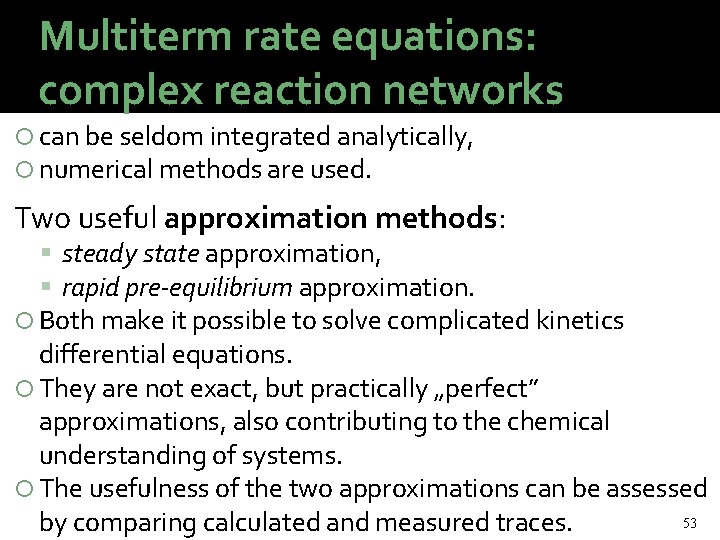
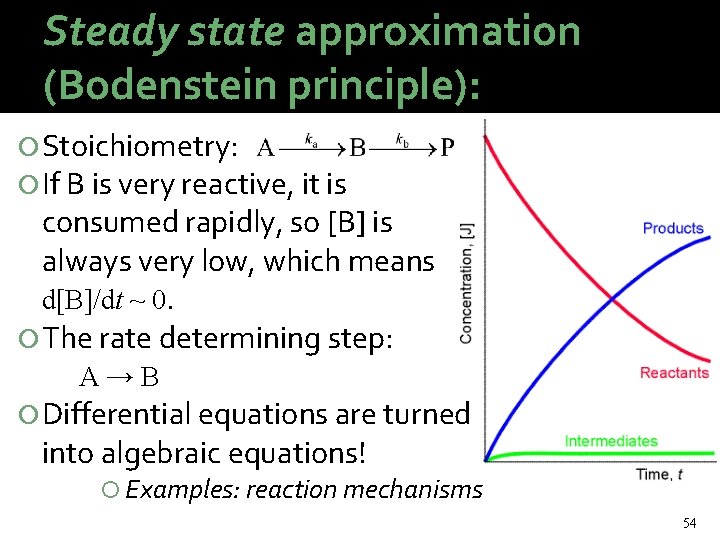
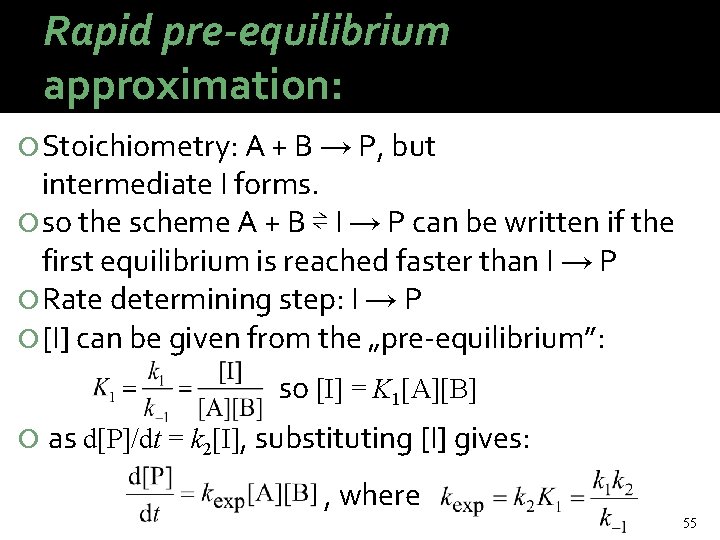
![Determining the rate equation: Rate equation: v = dc/dt = k[A]α[B]β[C]γ 1. Determination of Determining the rate equation: Rate equation: v = dc/dt = k[A]α[B]β[C]γ 1. Determination of](https://slidetodoc.com/presentation_image_h2/81d4bb159f6fcac9231140af0e61230c/image-56.jpg)
![Determining the rate equation: Rate equation: v = dc/dt = k[A]α[B]β[C]γ 1. Determination of Determining the rate equation: Rate equation: v = dc/dt = k[A]α[B]β[C]γ 1. Determination of](https://slidetodoc.com/presentation_image_h2/81d4bb159f6fcac9231140af0e61230c/image-57.jpg)
![Determining the rate equation: Rate equation: v = dc/dt = k[A]α[B]β[C]γ 1. Determination of Determining the rate equation: Rate equation: v = dc/dt = k[A]α[B]β[C]γ 1. Determination of](https://slidetodoc.com/presentation_image_h2/81d4bb159f6fcac9231140af0e61230c/image-58.jpg)
![Determining the rate equation: Rate equation: v = dc/dt = k[A]α[B]β[C]γ 1. Determination of Determining the rate equation: Rate equation: v = dc/dt = k[A]α[B]β[C]γ 1. Determination of](https://slidetodoc.com/presentation_image_h2/81d4bb159f6fcac9231140af0e61230c/image-59.jpg)
![Determining the rate equation: Rate equation: v = dc/dt = k[A]α[B]β[C]γ 1. Determination of Determining the rate equation: Rate equation: v = dc/dt = k[A]α[B]β[C]γ 1. Determination of](https://slidetodoc.com/presentation_image_h2/81d4bb159f6fcac9231140af0e61230c/image-60.jpg)
![Determining the rate equation: Rate equation: v = dc/dt = k[A]α[B]β[C]γ 1. Determination of Determining the rate equation: Rate equation: v = dc/dt = k[A]α[B]β[C]γ 1. Determination of](https://slidetodoc.com/presentation_image_h2/81d4bb159f6fcac9231140af0e61230c/image-61.jpg)
- Slides: 61
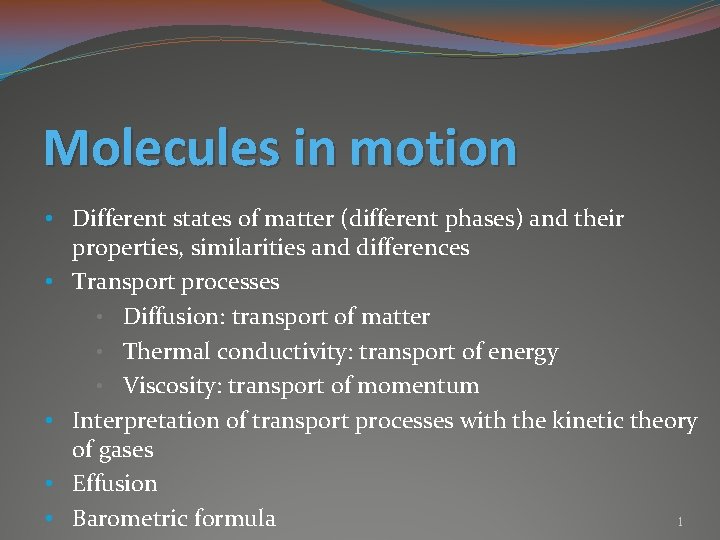
Molecules in motion • Different states of matter (different phases) and their properties, similarities and differences • Transport processes • Diffusion: transport of matter • Thermal conductivity: transport of energy • Viscosity: transport of momentum • Interpretation of transport processes with the kinetic theory of gases • Effusion • Barometric formula 1
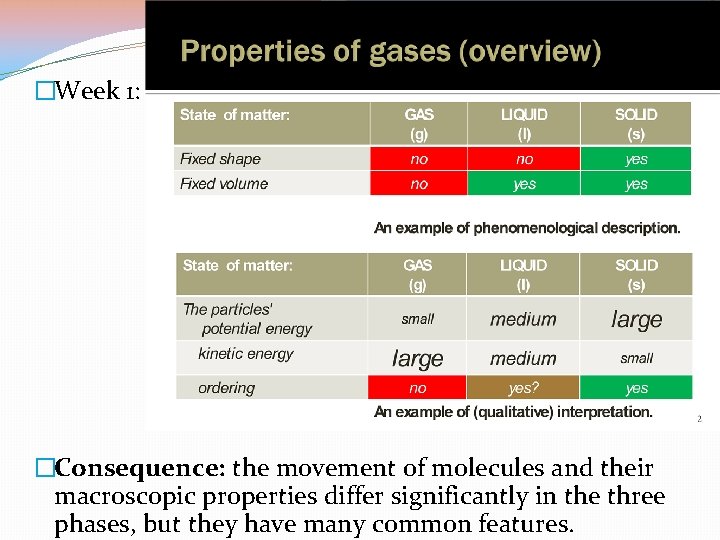
�Week 1: �Consequence: the movement of molecules and their macroscopic properties differ significantly in the three phases, but they have many common features.
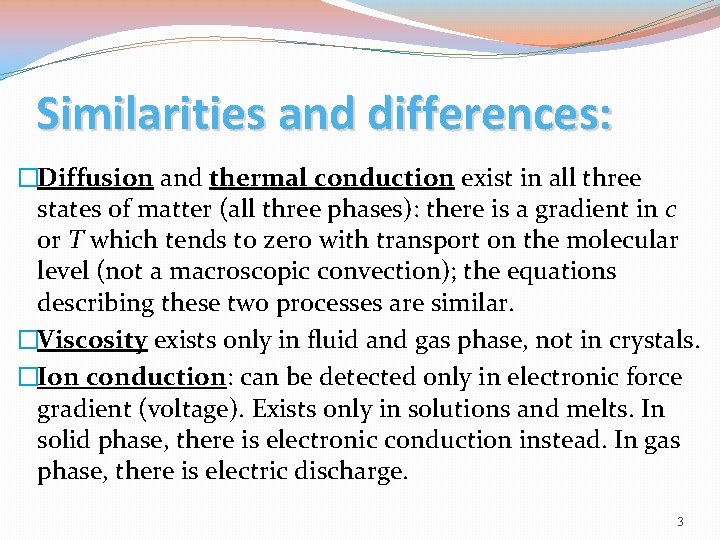
Similarities and differences: �Diffusion and thermal conduction exist in all three states of matter (all three phases): there is a gradient in c or T which tends to zero with transport on the molecular level (not a macroscopic convection); the equations describing these two processes are similar. �Viscosity exists only in fluid and gas phase, not in crystals. �Ion conduction: can be detected only in electronic force gradient (voltage). Exists only in solutions and melts. In solid phase, there is electronic conduction instead. In gas phase, there is electric discharge. 3
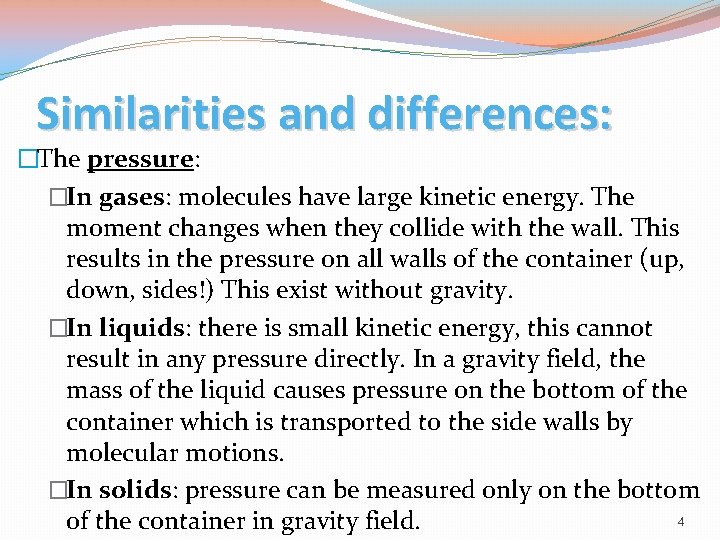
Similarities and differences: �The pressure: �In gases: molecules have large kinetic energy. The moment changes when they collide with the wall. This results in the pressure on all walls of the container (up, down, sides!) This exist without gravity. �In liquids: there is small kinetic energy, this cannot result in any pressure directly. In a gravity field, the mass of the liquid causes pressure on the bottom of the container which is transported to the side walls by molecular motions. �In solids: pressure can be measured only on the bottom 4 of the container in gravity field.
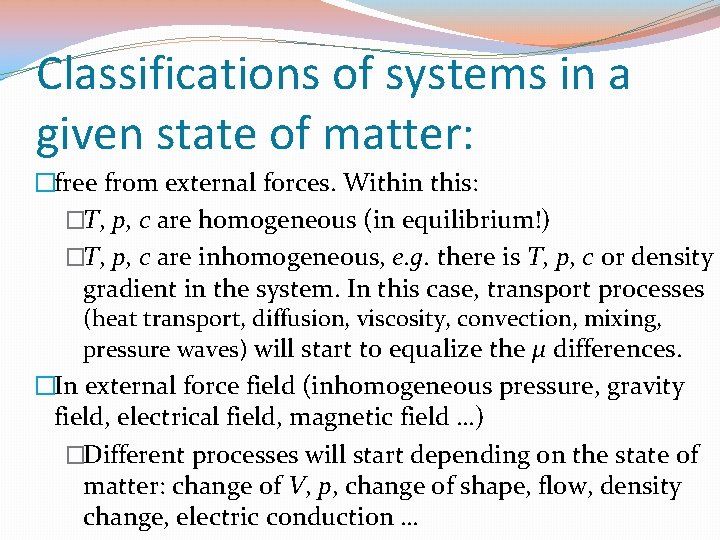
Classifications of systems in a given state of matter: �free from external forces. Within this: �T, p, c are homogeneous (in equilibrium!) �T, p, c are inhomogeneous, e. g. there is T, p, c or density gradient in the system. In this case, transport processes (heat transport, diffusion, viscosity, convection, mixing, pressure waves) will start to equalize the μ differences. �In external force field (inhomogeneous pressure, gravity field, electrical field, magnetic field …) �Different processes will start depending on the state of matter: change of V, p, change of shape, flow, density change, electric conduction …
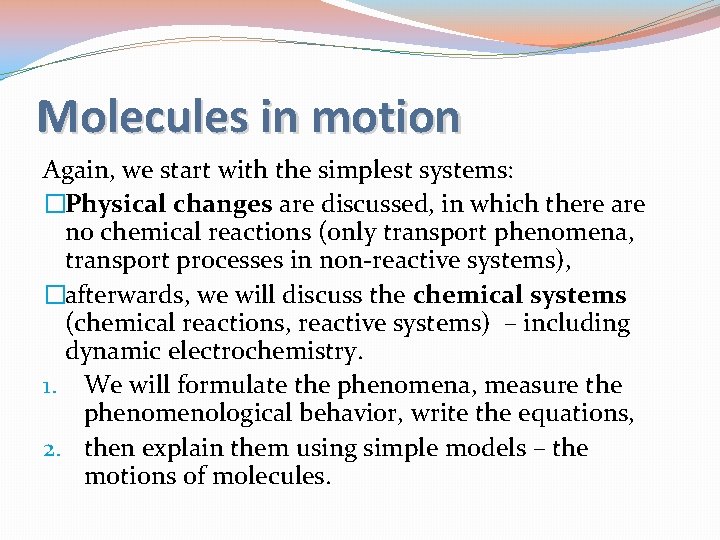
Molecules in motion Again, we start with the simplest systems: �Physical changes are discussed, in which there are no chemical reactions (only transport phenomena, transport processes in non-reactive systems), �afterwards, we will discuss the chemical systems (chemical reactions, reactive systems) – including dynamic electrochemistry. 1. We will formulate the phenomena, measure the phenomenological behavior, write the equations, 2. then explain them using simple models – the motions of molecules.
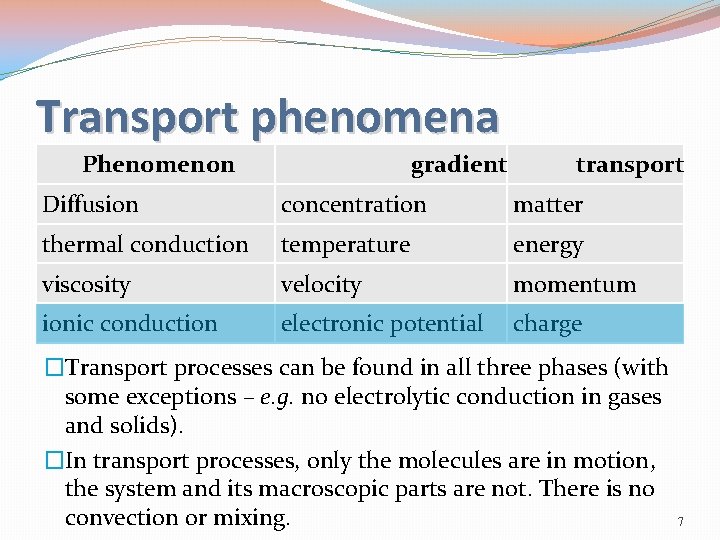
Transport phenomena Phenomenon gradient transport Diffusion concentration matter thermal conduction temperature energy viscosity velocity momentum ionic conduction electronic potential charge �Transport processes can be found in all three phases (with some exceptions – e. g. no electrolytic conduction in gases and solids). �In transport processes, only the molecules are in motion, the system and its macroscopic parts are not. There is no convection or mixing. 7
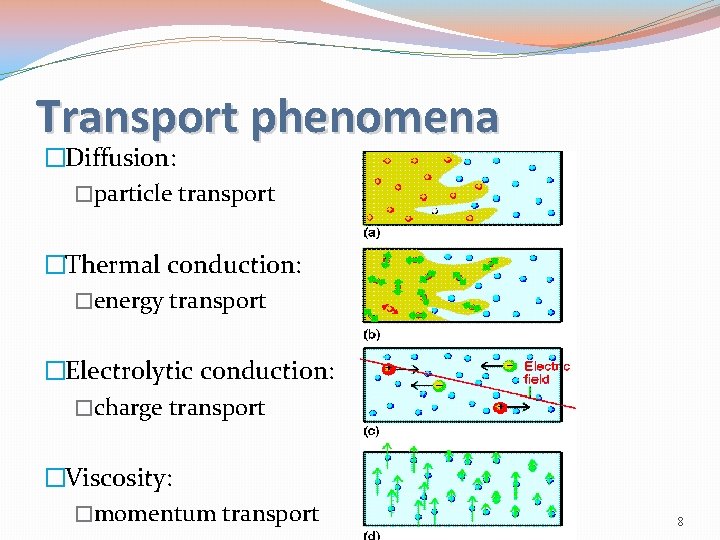
Transport phenomena �Diffusion: �particle transport �Thermal conduction: �energy transport �Electrolytic conduction: �charge transport �Viscosity: �momentum transport 8
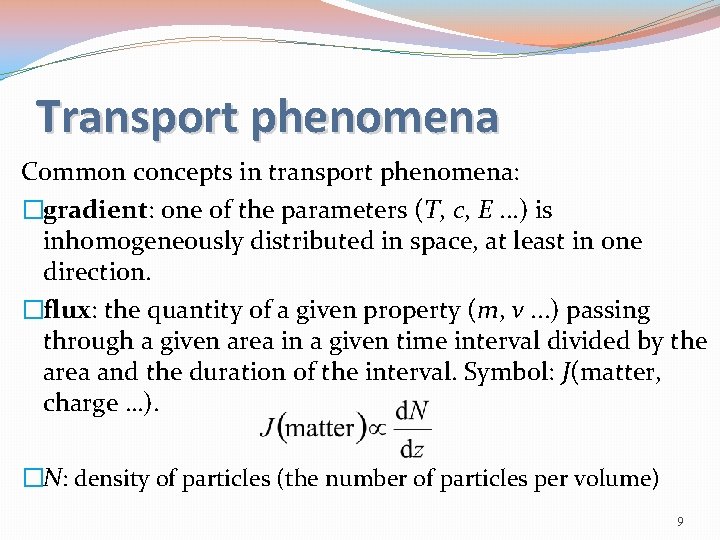
Transport phenomena Common concepts in transport phenomena: �gradient: one of the parameters (T, c, E. . . ) is inhomogeneously distributed in space, at least in one direction. �flux: the quantity of a given property (m, v. . . ) passing through a given area in a given time interval divided by the area and the duration of the interval. Symbol: J(matter, charge …). �N: density of particles (the number of particles per volume) 9
![Diffusion transport of matter at molecular level J m2 s1 D m 2 s1 Diffusion: transport of matter (at molecular level) [J]: m-2 s-1 [D]: m 2 s-1](https://slidetodoc.com/presentation_image_h2/81d4bb159f6fcac9231140af0e61230c/image-10.jpg)
Diffusion: transport of matter (at molecular level) [J]: m-2 s-1 [D]: m 2 s-1 [d. N/dz]: m-4 flux of matter diffusion coefficient concentration gradient �Fick’s first law of diffusion: diffusion will be faster when the concentration varies steeply with position than when the concentration is nearly uniform. �Different concentrations mean different chemical potentials (since μ depends on c), �Practical importance: e. g. motion of matter in soils. �Convection: macroscopic! 10
![Thermal conduction transport of energy J κ J m2 s1 flux of energy J Thermal conduction: transport of energy [J]: [κ]: J m-2 s-1 flux of energy J](https://slidetodoc.com/presentation_image_h2/81d4bb159f6fcac9231140af0e61230c/image-11.jpg)
Thermal conduction: transport of energy [J]: [κ]: J m-2 s-1 flux of energy J K-1 m-1 s-1 coefficient of thermal conductivity [d. T/dz]: K m-1 temperature gradient �Energy migrates down a temperature gradient. �The connection between flux and gradient is similar to Fick’s first law of diffusion. �Good thermal conductors: metals (Ag, Cu, Al), marble, diamond �Good thermal insulators: vacuum, CO 2, plastic, wood �Practical importance: thermal insulation of houses. �There is molecular heat conduction, macroscopic 11 (convective) heat flow and heat radiation.
![Viscosity transport of momentum J η kg m1 s2 kg m1 s1 flux of Viscosity: transport of momentum [J]: [η]: kg m-1 s-2 kg m-1 s-1 flux of](https://slidetodoc.com/presentation_image_h2/81d4bb159f6fcac9231140af0e61230c/image-12.jpg)
Viscosity: transport of momentum [J]: [η]: kg m-1 s-2 kg m-1 s-1 flux of momentum coefficient of viscosity (or simply ‘the viscosity’) 1 P (poise) = 0. 1 kg m-1 s-1 [dvx/dz]: s-1 velocity gradient �Because the retarding effect depends on the transfer of the xcomponent of linear momentum into the layer of interest, �the viscosity depends on the flux of this x-component in the zdirection. 12
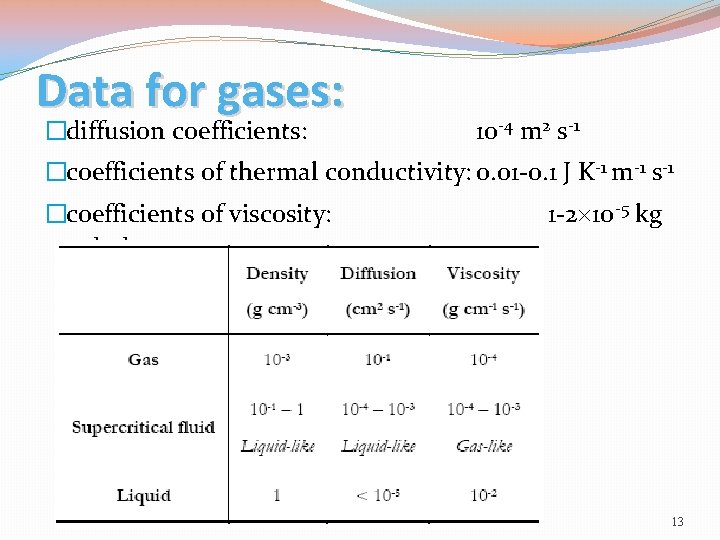
Data for gases: �diffusion coefficients: 10 -4 m 2 s-1 �coefficients of thermal conductivity: 0. 01 -0. 1 J K-1 m-1 s-1 �coefficients of viscosity: m-1 s-1 1 -2 10 -5 kg 13
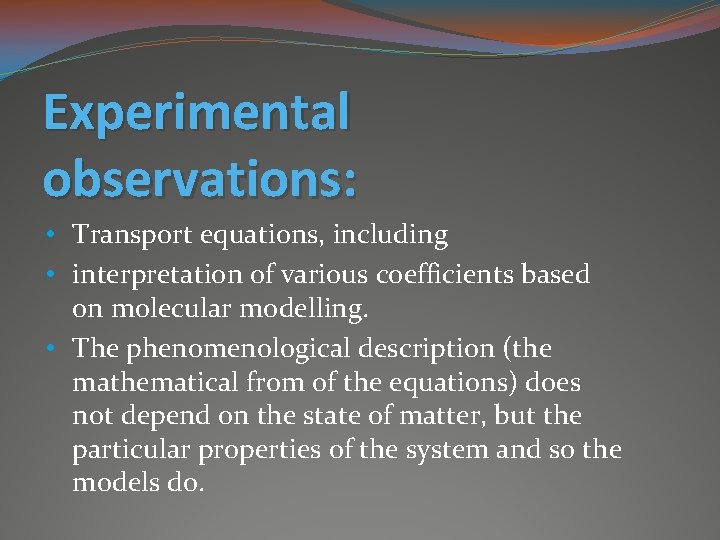
Experimental observations: • Transport equations, including • interpretation of various coefficients based on molecular modelling. • The phenomenological description (the mathematical from of the equations) does not depend on the state of matter, but the particular properties of the system and so the models do.
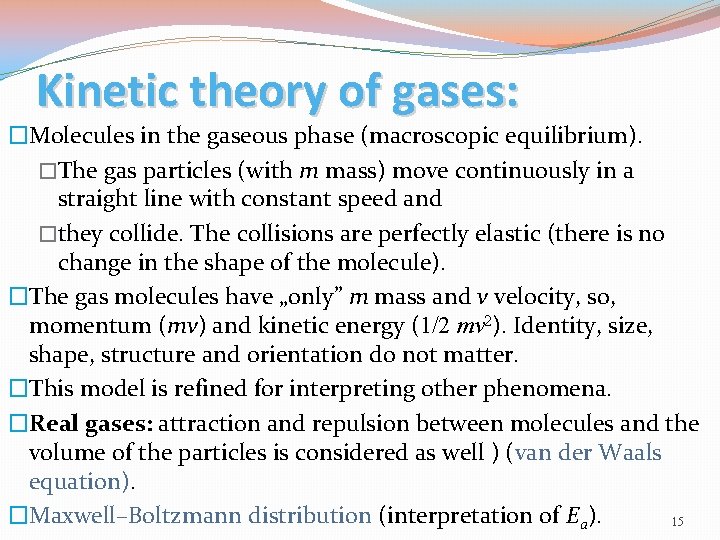
Kinetic theory of gases: �Molecules in the gaseous phase (macroscopic equilibrium). �The gas particles (with m mass) move continuously in a straight line with constant speed and �they collide. The collisions are perfectly elastic (there is no change in the shape of the molecule). �The gas molecules have „only” m mass and v velocity, so, momentum (mv) and kinetic energy (1/2 mv 2). Identity, size, shape, structure and orientation do not matter. �This model is refined for interpreting other phenomena. �Real gases: attraction and repulsion between molecules and the volume of the particles is considered as well ) (van der Waals equation). �Maxwell–Boltzmann distribution (interpretation of Ea). 15
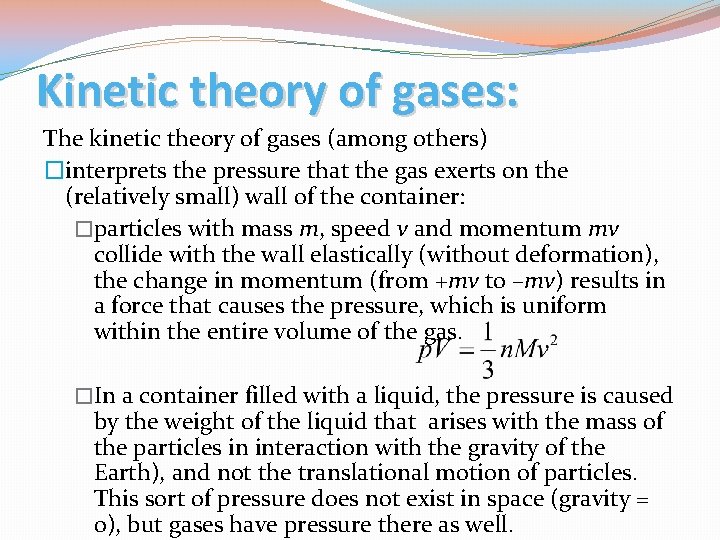
Kinetic theory of gases: The kinetic theory of gases (among others) �interprets the pressure that the gas exerts on the (relatively small) wall of the container: �particles with mass m, speed v and momentum mv collide with the wall elastically (without deformation), the change in momentum (from +mv to –mv) results in a force that causes the pressure, which is uniform within the entire volume of the gas. �In a container filled with a liquid, the pressure is caused by the weight of the liquid that arises with the mass of the particles in interaction with the gravity of the Earth), and not the translational motion of particles. This sort of pressure does not exist in space (gravity = 0), but gases have pressure there as well.
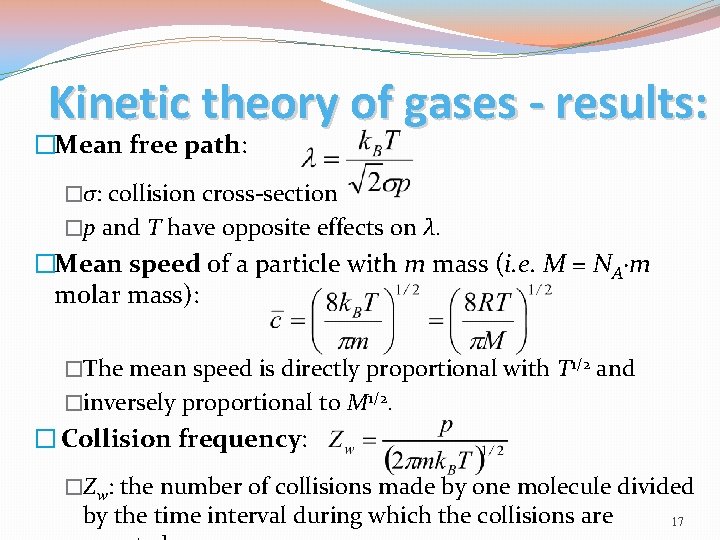
Kinetic theory of gases - results: �Mean free path: �σ: collision cross-section �p and T have opposite effects on λ. �Mean speed of a particle with m mass (i. e. M = NA·m molar mass): �The mean speed is directly proportional with T 1/2 and �inversely proportional to M 1/2. � Collision frequency: �Zw: the number of collisions made by one molecule divided by the time interval during which the collisions are 17
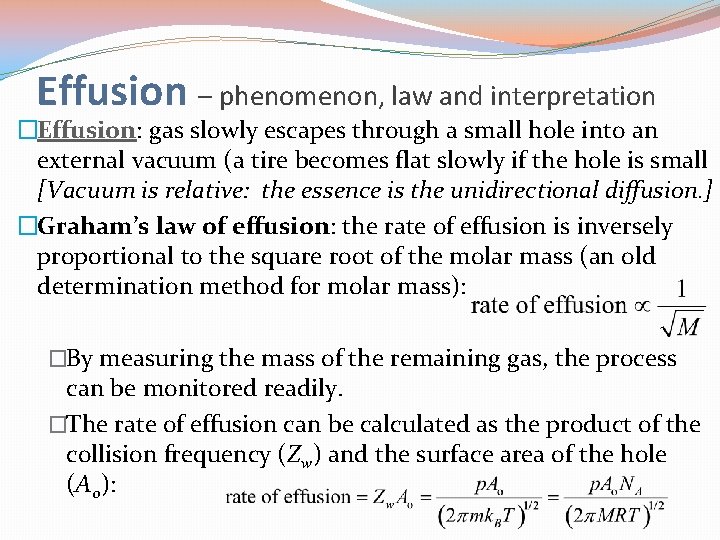
Effusion – phenomenon, law and interpretation �Effusion: gas slowly escapes through a small hole into an external vacuum (a tire becomes flat slowly if the hole is small [Vacuum is relative: the essence is the unidirectional diffusion. ] �Graham’s law of effusion: the rate of effusion is inversely proportional to the square root of the molar mass (an old determination method for molar mass): �By measuring the mass of the remaining gas, the process can be monitored readily. �The rate of effusion can be calculated as the product of the collision frequency (Zw) and the surface area of the hole (A 0):
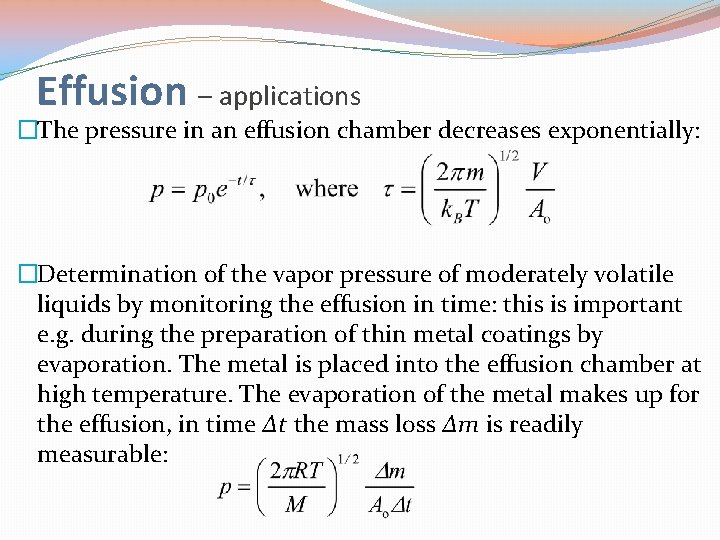
Effusion – applications �The pressure in an effusion chamber decreases exponentially: �Determination of the vapor pressure of moderately volatile liquids by monitoring the effusion in time: this is important e. g. during the preparation of thin metal coatings by evaporation. The metal is placed into the effusion chamber at high temperature. The evaporation of the metal makes up for the effusion, in time Δt the mass loss Δm is readily measurable:
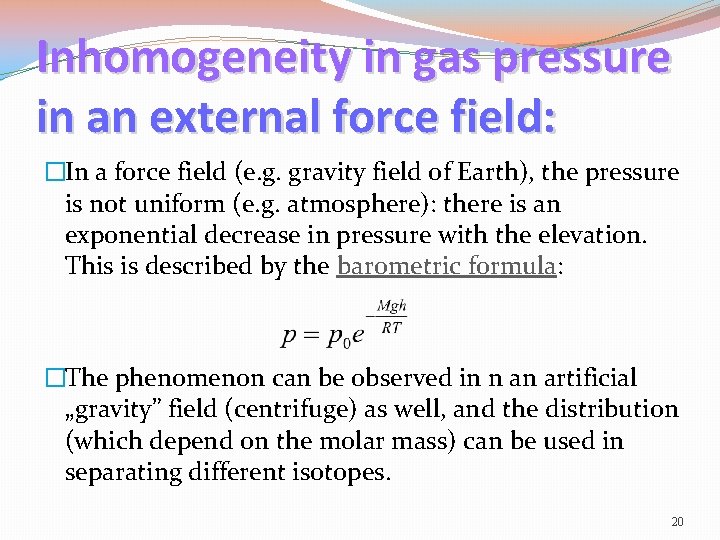
Inhomogeneity in gas pressure in an external force field: �In a force field (e. g. gravity field of Earth), the pressure is not uniform (e. g. atmosphere): there is an exponential decrease in pressure with the elevation. This is described by the barometric formula: �The phenomenon can be observed in n an artificial „gravity” field (centrifuge) as well, and the distribution (which depend on the molar mass) can be used in separating different isotopes. 20
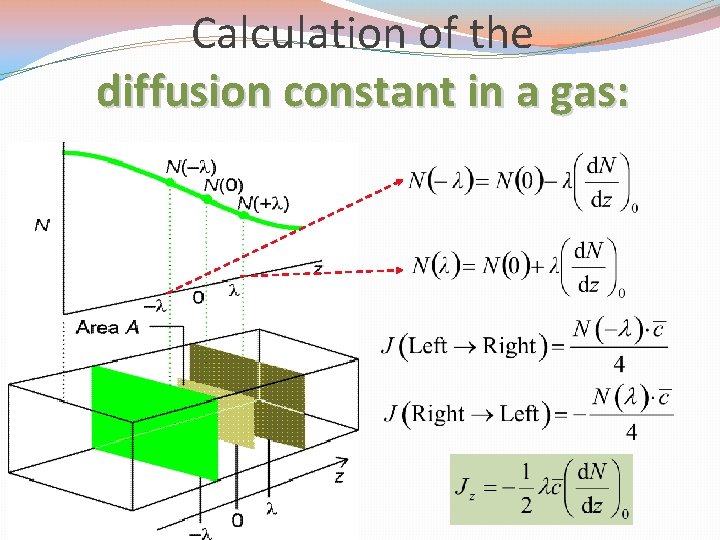
Calculation of the diffusion constant in a gas:
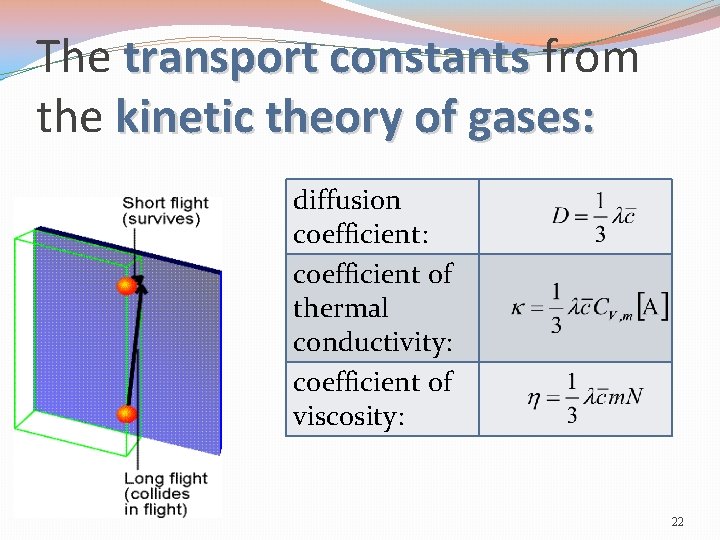
The transport constants from the kinetic theory of gases: diffusion coefficient: coefficient of thermal conductivity: coefficient of viscosity: 22
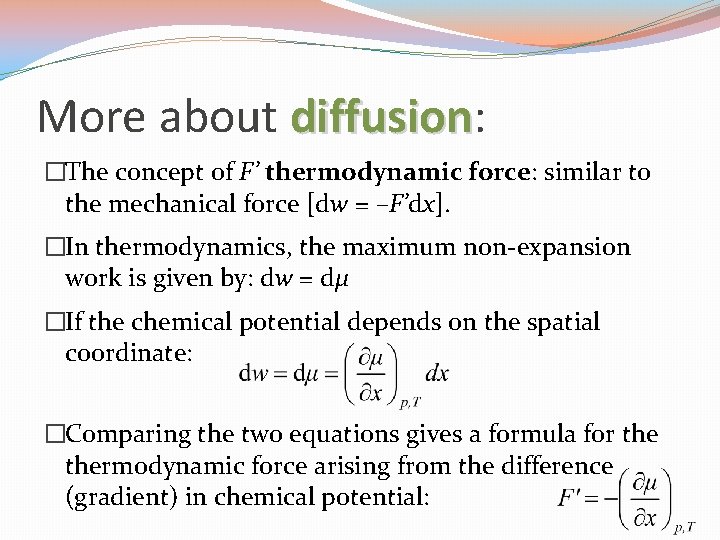
More about diffusion: diffusion �The concept of F’ thermodynamic force: similar to the mechanical force [dw = –F’dx]. �In thermodynamics, the maximum non-expansion work is given by: dw = dμ �If the chemical potential depends on the spatial coordinate: �Comparing the two equations gives a formula for thermodynamic force arising from the difference (gradient) in chemical potential:
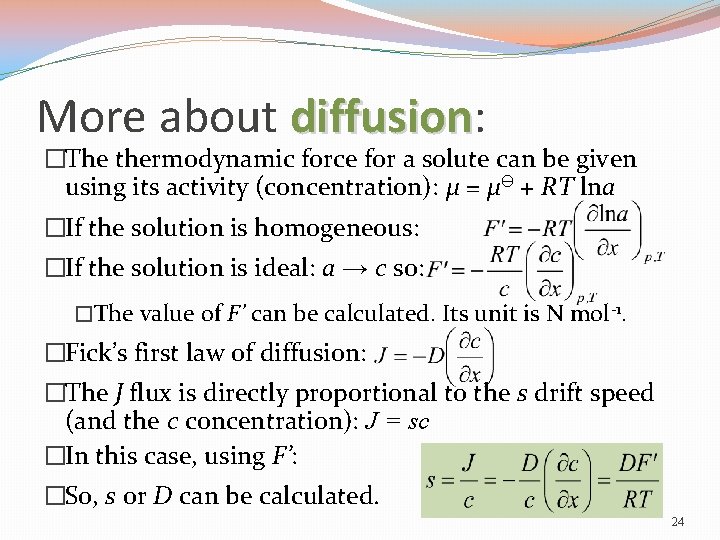
More about diffusion: diffusion �The thermodynamic force for a solute can be given using its activity (concentration): μ = μӨ + RT lna �If the solution is homogeneous: �If the solution is ideal: a → c so: �The value of F’ can be calculated. Its unit is N mol-1. �Fick’s first law of diffusion: �The J flux is directly proportional to the s drift speed (and the c concentration): J = sc �In this case, using F’: �So, s or D can be calculated. 24
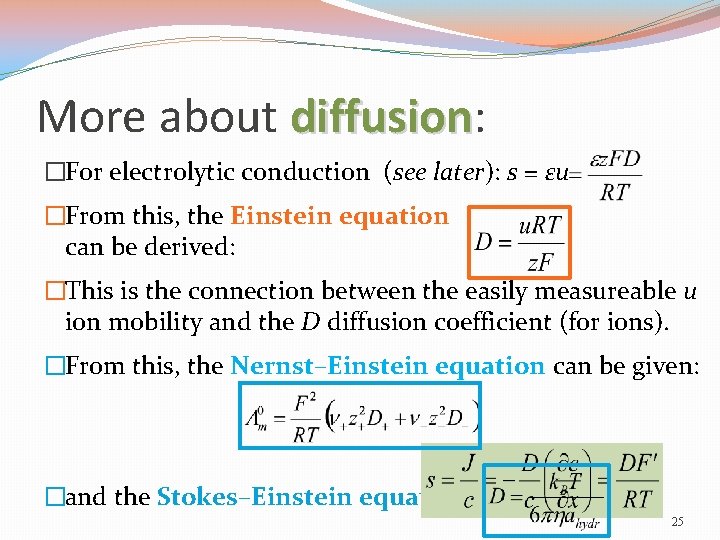
More about diffusion: diffusion �For electrolytic conduction (see later): s = εu �From this, the Einstein equation can be derived: �This is the connection between the easily measureable u ion mobility and the D diffusion coefficient (for ions). �From this, the Nernst–Einstein equation can be given: �and the Stokes–Einstein equation: 25
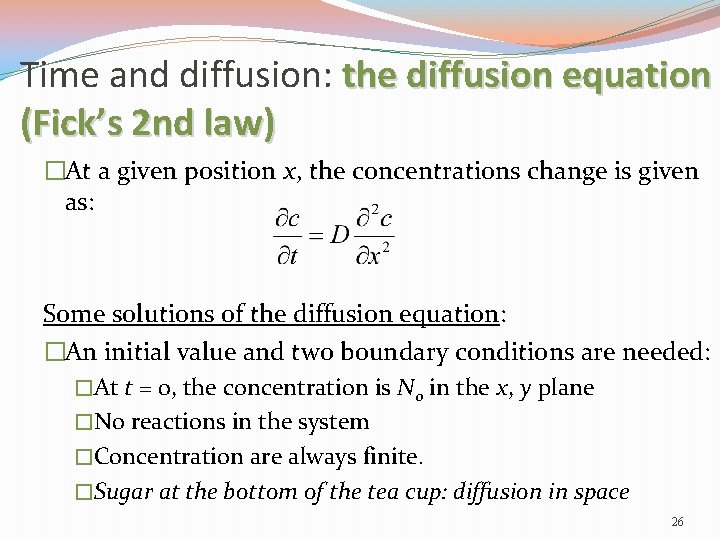
Time and diffusion: the diffusion equation (Fick’s 2 nd law) �At a given position x, the concentrations change is given as: Some solutions of the diffusion equation: �An initial value and two boundary conditions are needed: �At t = 0, the concentration is N 0 in the x, y plane �No reactions in the system �Concentration are always finite. �Sugar at the bottom of the tea cup: diffusion in space 26
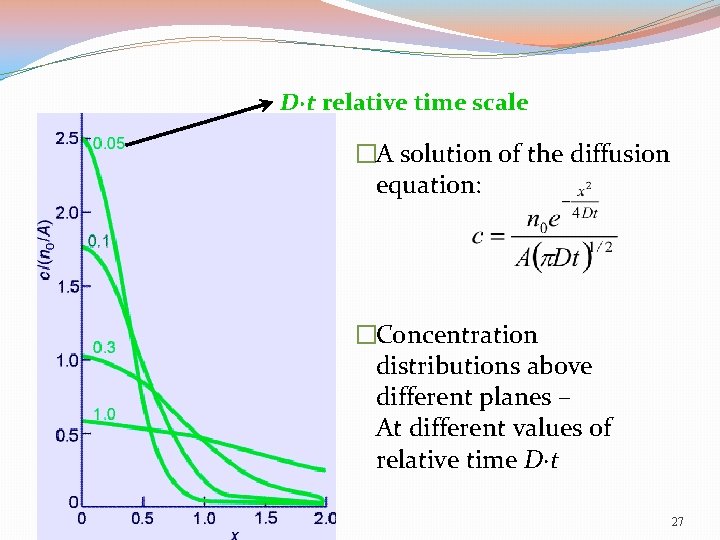
D·t relative time scale �A solution of the diffusion equation: �Concentration distributions above different planes – At different values of relative time D·t 27
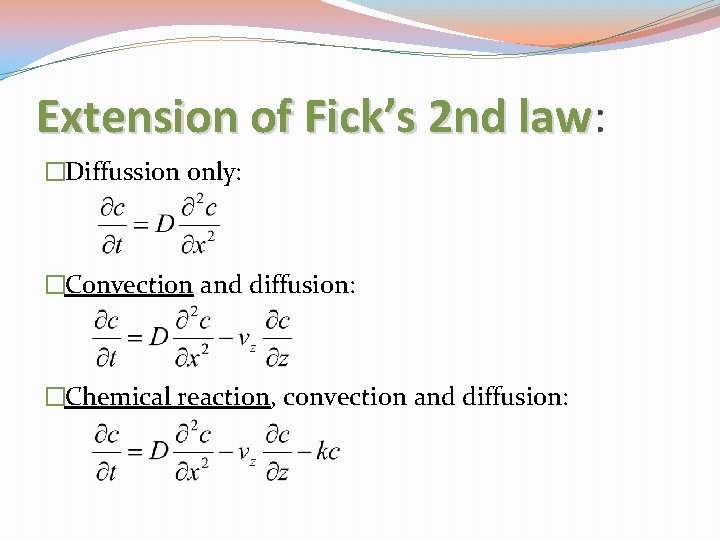
Extension of Fick’s 2 nd law: law �Diffussion only: �Convection and diffusion: �Chemical reaction, convection and diffusion:
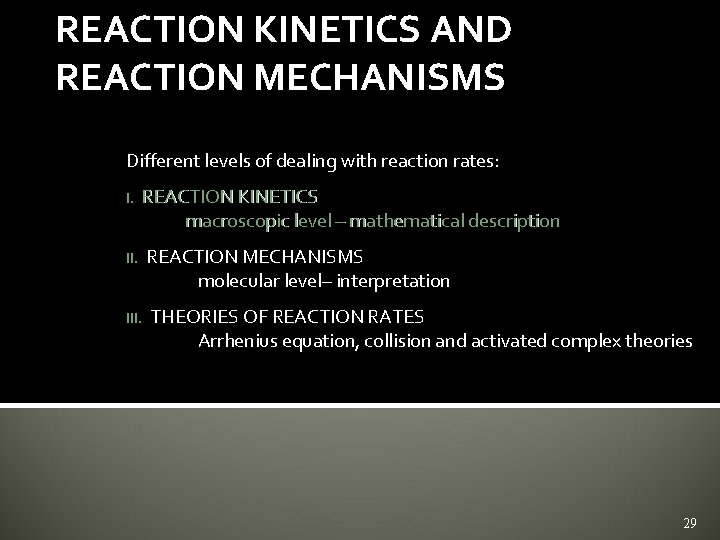
REACTION KINETICS AND REACTION MECHANISMS Different levels of dealing with reaction rates: I. REACTION KINETICS macroscopic level – mathematical description II. REACTION MECHANISMS molecular level– interpretation III. THEORIES OF REACTION RATES Arrhenius equation, collision and activated complex theories 29
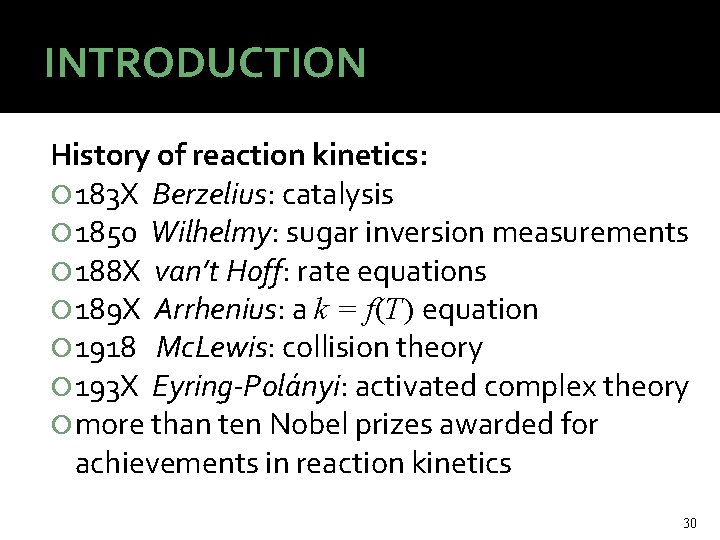
INTRODUCTION History of reaction kinetics: 183 X Berzelius: catalysis 1850 Wilhelmy: sugar inversion measurements 188 X van’t Hoff: rate equations 189 X Arrhenius: a k = f(T) equation 1918 Mc. Lewis: collision theory 193 X Eyring-Polányi: activated complex theory more than ten Nobel prizes awarded for achievements in reaction kinetics 30
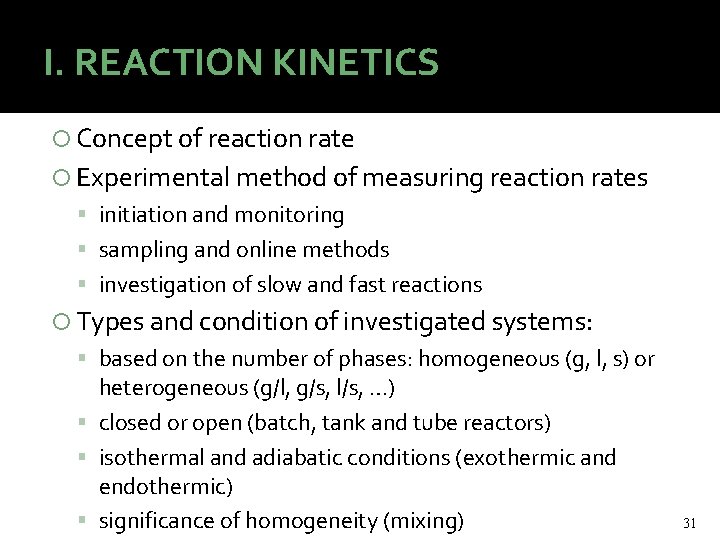
I. REACTION KINETICS Concept of reaction rate Experimental method of measuring reaction rates initiation and monitoring sampling and online methods investigation of slow and fast reactions Types and condition of investigated systems: based on the number of phases: homogeneous (g, l, s) or heterogeneous (g/l, g/s, l/s, …) closed or open (batch, tank and tube reactors) isothermal and adiabatic conditions (exothermic and endothermic) significance of homogeneity (mixing) 31
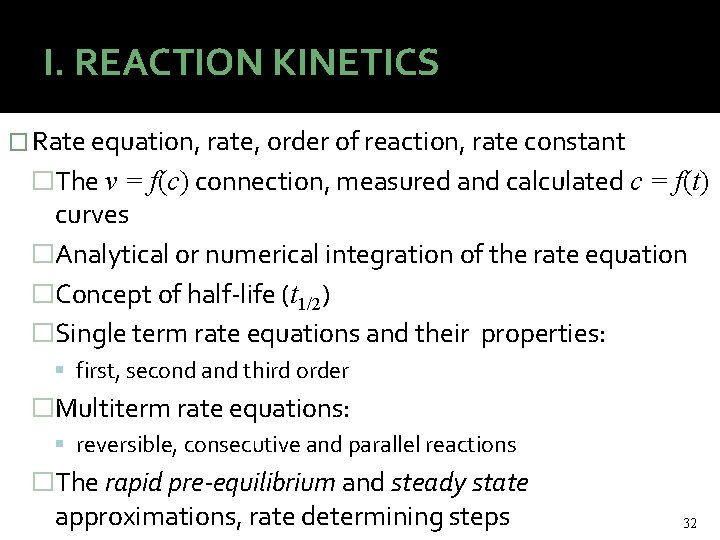
I. REACTION KINETICS � Rate equation, rate, order of reaction, rate constant �The v = f(c) connection, measured and calculated c = f(t) curves �Analytical or numerical integration of the rate equation �Concept of half-life (t 1/2) �Single term rate equations and their properties: first, second and third order �Multiterm rate equations: reversible, consecutive and parallel reactions �The rapid pre-equilibrium and steady state approximations, rate determining steps 32
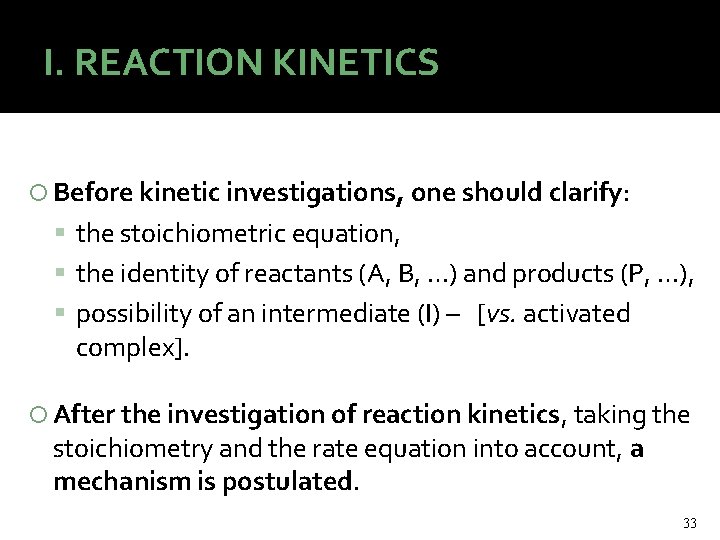
I. REACTION KINETICS Before kinetic investigations, one should clarify: the stoichiometric equation, the identity of reactants (A, B, …) and products (P, …), possibility of an intermediate (I) – [vs. activated complex]. After the investigation of reaction kinetics, taking the stoichiometry and the rate equation into account, a mechanism is postulated. 33
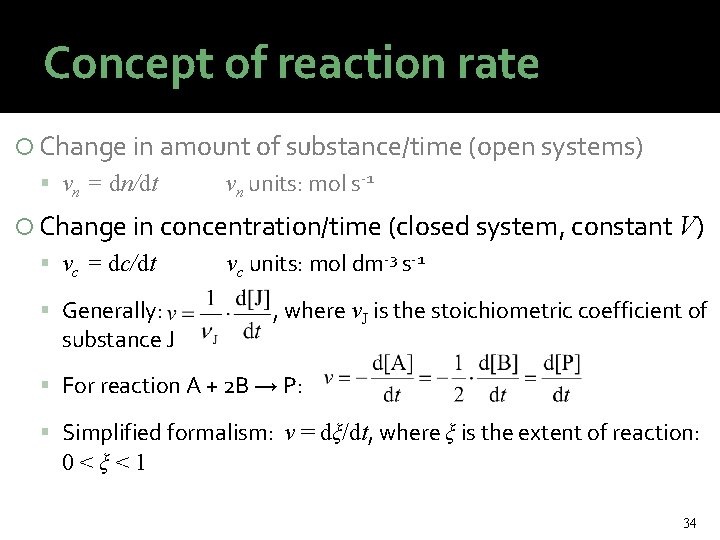
Concept of reaction rate Change in amount of substance/time (open systems) vn = dn/dt vn units: mol s-1 Change in concentration/time (closed system, constant V) vc = dc/dt Generally: substance J vc units: mol dm-3 s-1 , where νJ is the stoichiometric coefficient of For reaction A + 2 B → P: Simplified formalism: v = dξ/dt, where ξ is the extent of reaction: 0<ξ<1 34
![Visualizing the reaction rate graphical representation Rate equation v dcdt kAαBβCγ Visualizing the reaction rate – graphical representation Rate equation: v = dc/dt = k[A]α[B]β[C]γ…](https://slidetodoc.com/presentation_image_h2/81d4bb159f6fcac9231140af0e61230c/image-35.jpg)
Visualizing the reaction rate – graphical representation Rate equation: v = dc/dt = k[A]α[B]β[C]γ… reactant: dc/dt negative product: dc/dt positive The rate of reaction at time t (or concentration c) is determined based on the slopes of the experimentally measured c – t (or c = f(t)) curves. 35
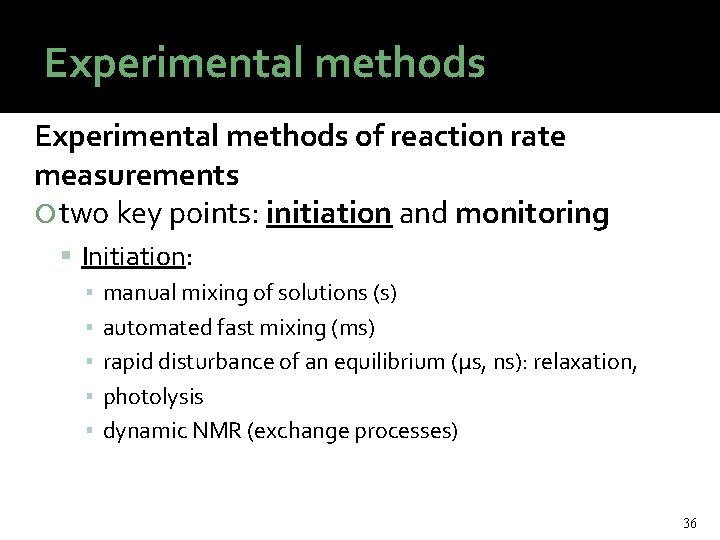
Experimental methods of reaction rate measurements two key points: initiation and monitoring Initiation: ▪ manual mixing of solutions (s) ▪ automated fast mixing (ms) ▪ rapid disturbance of an equilibrium (μs, ns): relaxation, ▪ photolysis ▪ dynamic NMR (exchange processes) 36
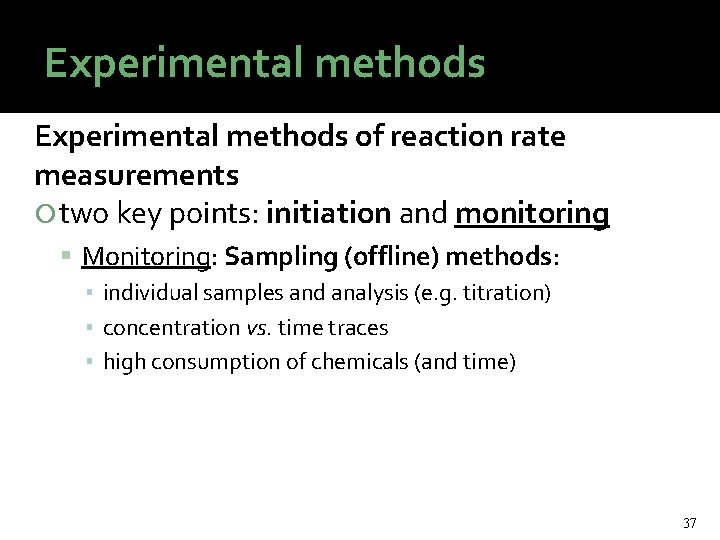
Experimental methods of reaction rate measurements two key points: initiation and monitoring Monitoring: Sampling (offline) methods: ▪ individual samples and analysis (e. g. titration) ▪ concentration vs. time traces ▪ high consumption of chemicals (and time) 37
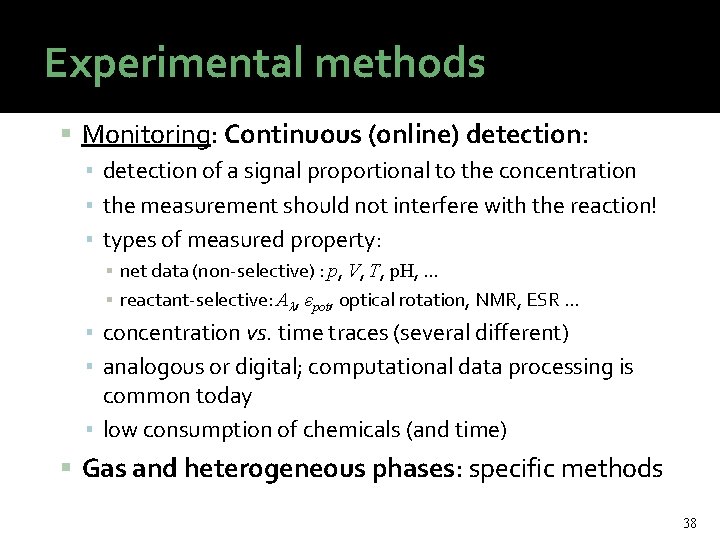
Experimental methods Monitoring: Continuous (online) detection: ▪ detection of a signal proportional to the concentration ▪ the measurement should not interfere with the reaction! ▪ types of measured property: ▪ net data (non-selective) : p, V, T, p. H, … ▪ reactant-selective: Aλ, εpot, optical rotation, NMR, ESR … ▪ concentration vs. time traces (several different) ▪ analogous or digital; computational data processing is common today ▪ low consumption of chemicals (and time) Gas and heterogeneous phases: specific methods 38
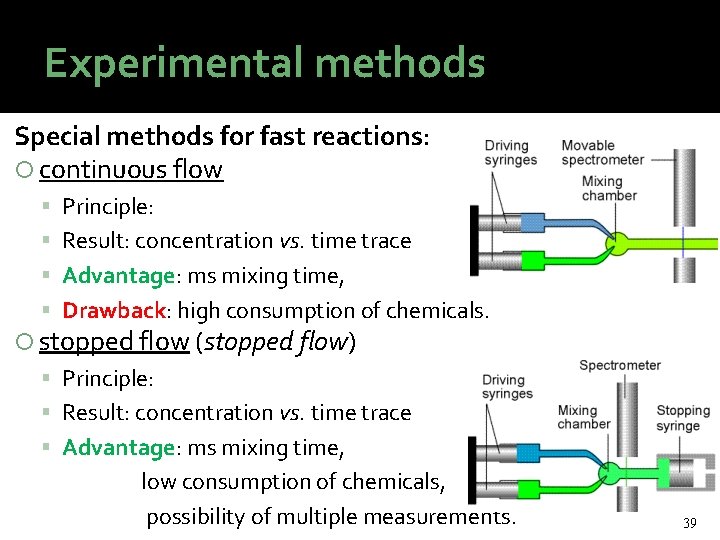
Experimental methods Special methods for fast reactions: continuous flow Principle: Result: concentration vs. time trace Advantage: ms mixing time, Drawback: high consumption of chemicals. stopped flow (stopped flow) Principle: Result: concentration vs. time trace Advantage: ms mixing time, low consumption of chemicals, possibility of multiple measurements. 39
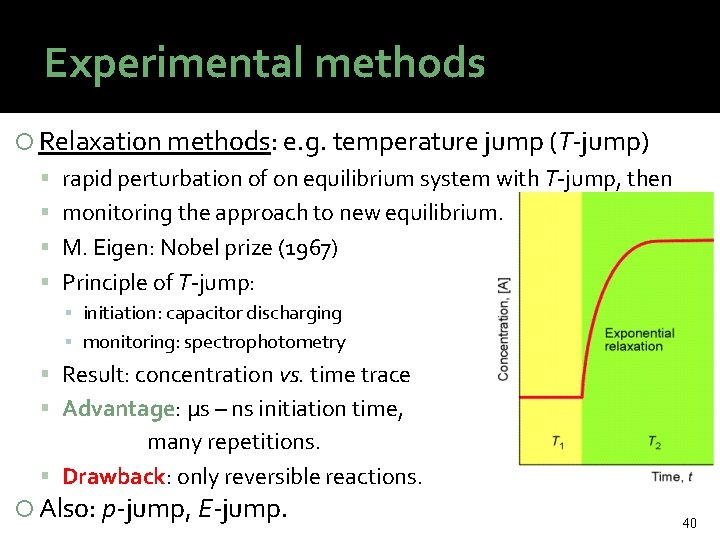
Experimental methods Relaxation methods: e. g. temperature jump (T-jump) rapid perturbation of on equilibrium system with T-jump, then monitoring the approach to new equilibrium. M. Eigen: Nobel prize (1967) Principle of T-jump: ▪ initiation: capacitor discharging ▪ monitoring: spectrophotometry Result: concentration vs. time trace Advantage: µs – ns initiation time, many repetitions. Drawback: only reversible reactions. Also: p-jump, E-jump. 40
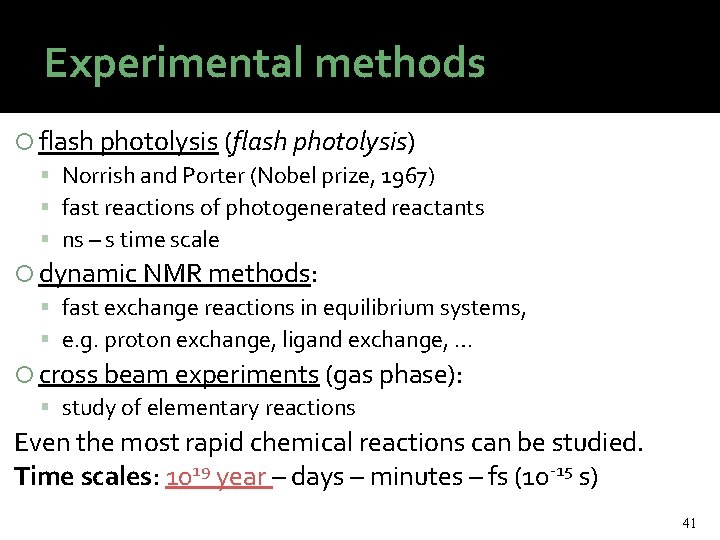
Experimental methods flash photolysis (flash photolysis) Norrish and Porter (Nobel prize, 1967) fast reactions of photogenerated reactants ns – s time scale dynamic NMR methods: fast exchange reactions in equilibrium systems, e. g. proton exchange, ligand exchange, … cross beam experiments (gas phase): study of elementary reactions Even the most rapid chemical reactions can be studied. Time scales: 1019 year – days – minutes – fs (10 -15 s) 41
![Rate equation v dcdt kAαBβCγ v rate of reaction k rate Rate equation � v = dc/dt = k[A]α[B]β[C]γ… v: rate of reaction k: rate](https://slidetodoc.com/presentation_image_h2/81d4bb159f6fcac9231140af0e61230c/image-42.jpg)
Rate equation � v = dc/dt = k[A]α[B]β[C]γ… v: rate of reaction k: rate constant (= rate coefficient) α, β, γ: order of reaction; α + β + γ: net order f reaction The rate equation is v – c (i. e. v = f(c)) connections, differential equation Measurement results: c – t traces Integration of the differential equation (analytical or numerical) is needed for comparison The reaction orders are decided based on the comparison of integrated forms and than the rate constant(s) are calculated 42
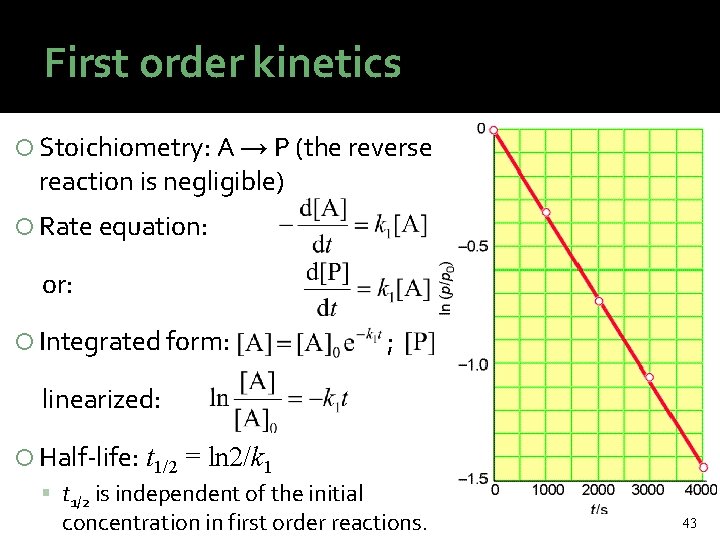
First order kinetics Stoichiometry: A → P (the reverse reaction is negligible) Rate equation: or: Integrated form: ; linearized: Half-life: t 1/2 = ln 2/k 1 t 1/2 is independent of the initial concentration in first order reactions. 43
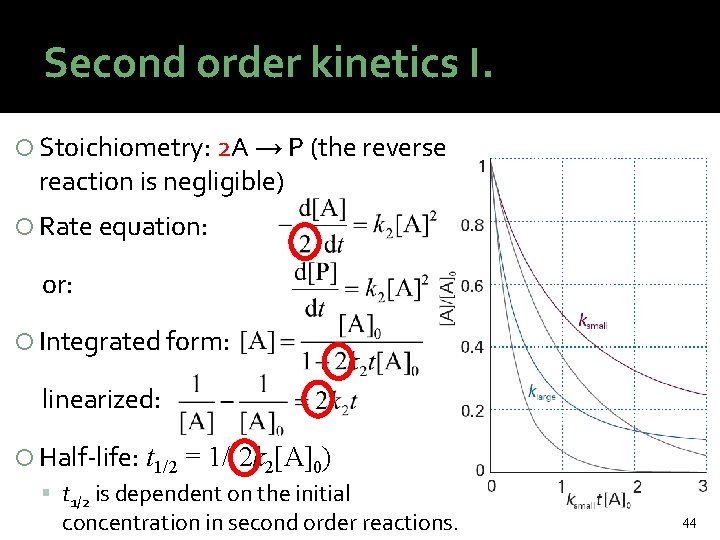
Second order kinetics I. Stoichiometry: 2 A → P (the reverse reaction is negligible) Rate equation: or: Integrated form: linearized: Half-life: t 1/2 = 1/(2 k 2[A]0) t 1/2 is dependent on the initial concentration in second order reactions. 44
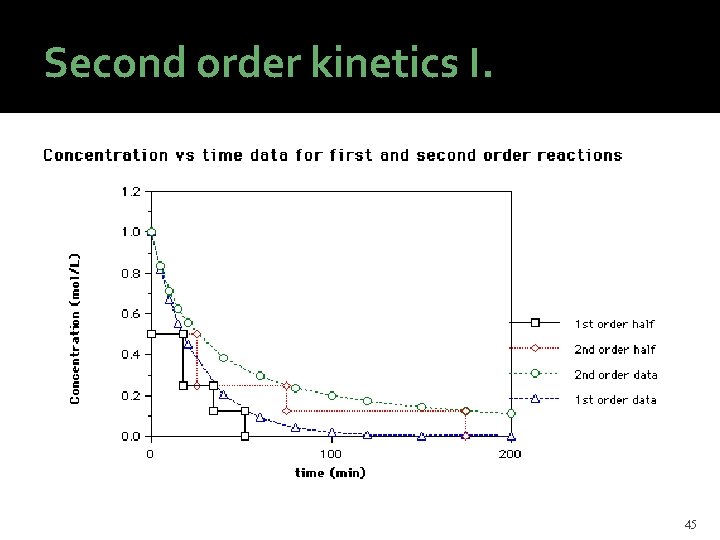
Second order kinetics I. 45
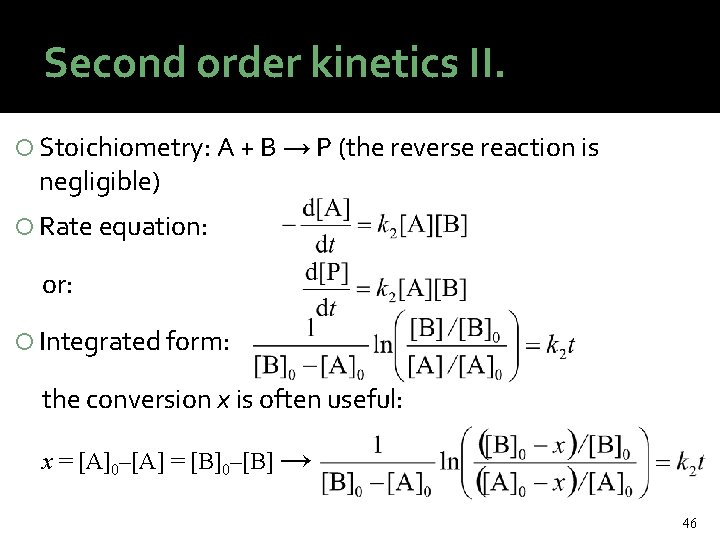
Second order kinetics II. Stoichiometry: A + B → P (the reverse reaction is negligible) Rate equation: or: Integrated form: the conversion x is often useful: x = [A]0–[A] = [B]0–[B] → 46
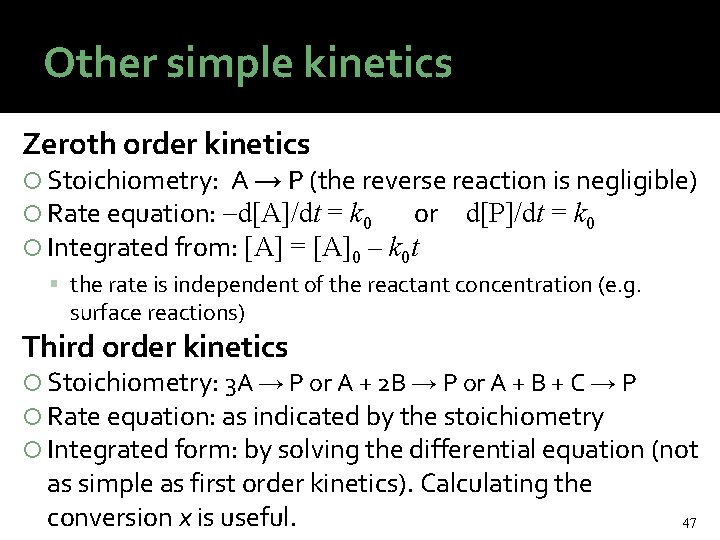
Other simple kinetics Zeroth order kinetics Stoichiometry: A → P (the reverse reaction is negligible) Rate equation: d[A]/dt = k 0 or d[P]/dt = k 0 Integrated from: [A] = [A]0 – k 0 t the rate is independent of the reactant concentration (e. g. surface reactions) Third order kinetics Stoichiometry: 3 A → P or A + 2 B → P or A + B + C → P Rate equation: as indicated by the stoichiometry Integrated form: by solving the differential equation (not as simple as first order kinetics). Calculating the conversion x is useful. 47
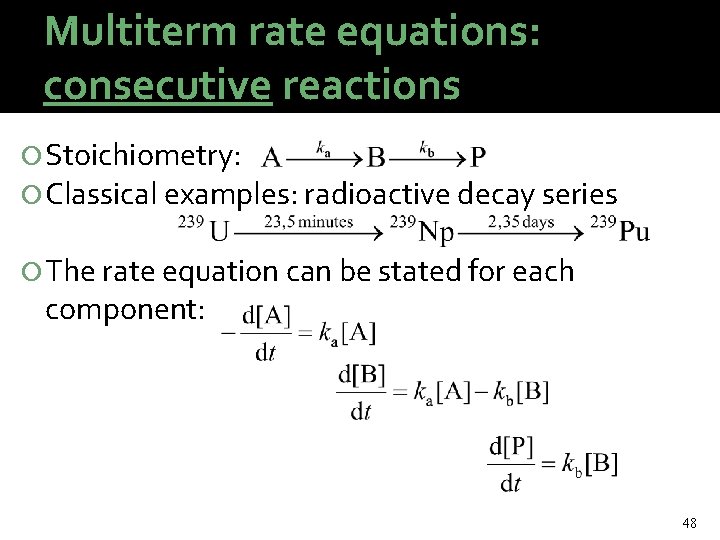
Multiterm rate equations: consecutive reactions Stoichiometry: Classical examples: radioactive decay series The rate equation can be stated for each component: 48
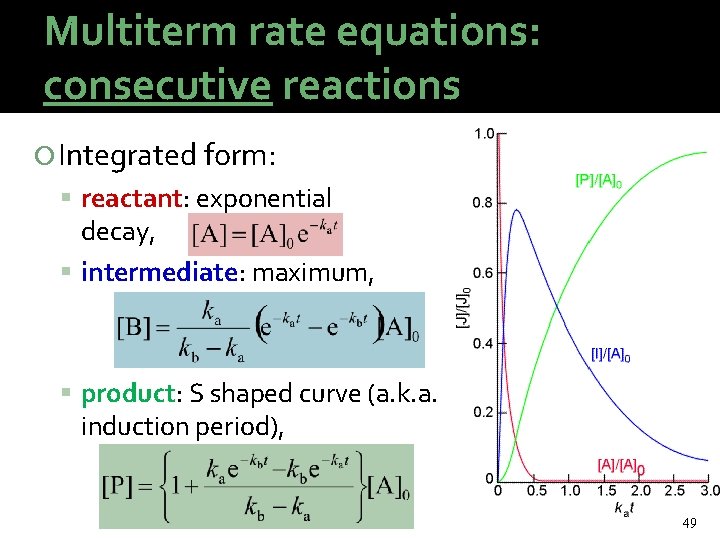
Multiterm rate equations: consecutive reactions Integrated form: reactant: exponential decay, intermediate: maximum, product: S shaped curve (a. k. a. induction period), 49
![Multiterm rate equations parallel reactions Relatively simple close to obvious Stoichiometry dP 1dt Multiterm rate equations: parallel reactions Relatively simple (close to obvious) Stoichiometry: d[P 1]/dt =](https://slidetodoc.com/presentation_image_h2/81d4bb159f6fcac9231140af0e61230c/image-50.jpg)
Multiterm rate equations: parallel reactions Relatively simple (close to obvious) Stoichiometry: d[P 1]/dt = k 1[A] d[P 2]/dt = k 2[A] d[Pi]/dt = ki[A] −d[A]/dt = (k 1 + k 2 + … + ki)[A] Integration gives exponential (first order) formulas. 50
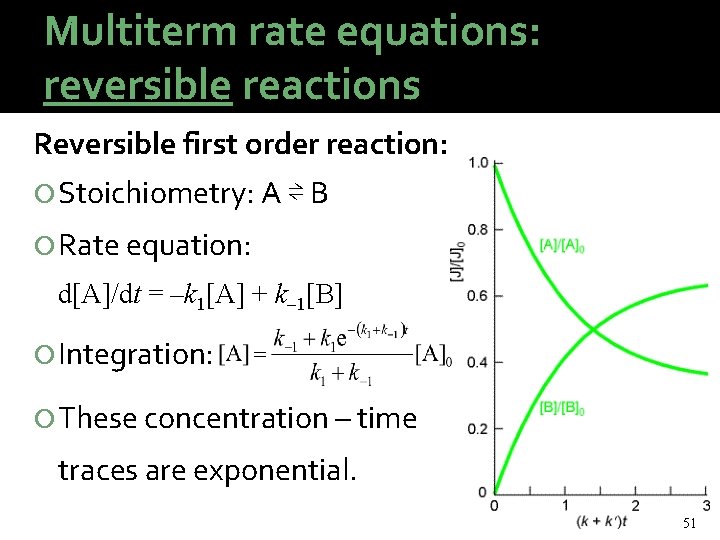
Multiterm rate equations: reversible reactions Reversible first order reaction: Stoichiometry: A ⇌ B Rate equation: d[A]/dt = –k 1[A] + k 1[B] Integration: These concentration – time traces are exponential. 51
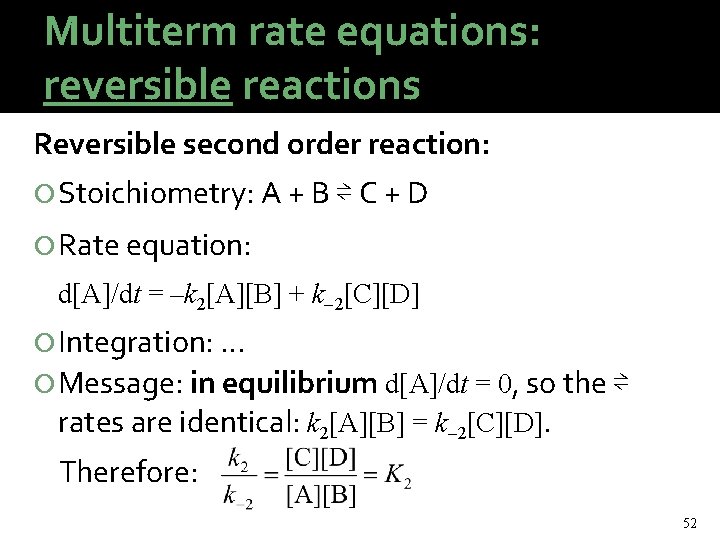
Multiterm rate equations: reversible reactions Reversible second order reaction: Stoichiometry: A + B ⇌ C + D Rate equation: d[A]/dt = –k 2[A][B] + k 2[C][D] Integration: … Message: in equilibrium d[A]/dt = 0, so the ⇌ rates are identical: k 2[A][B] = k 2[C][D]. Therefore: 52
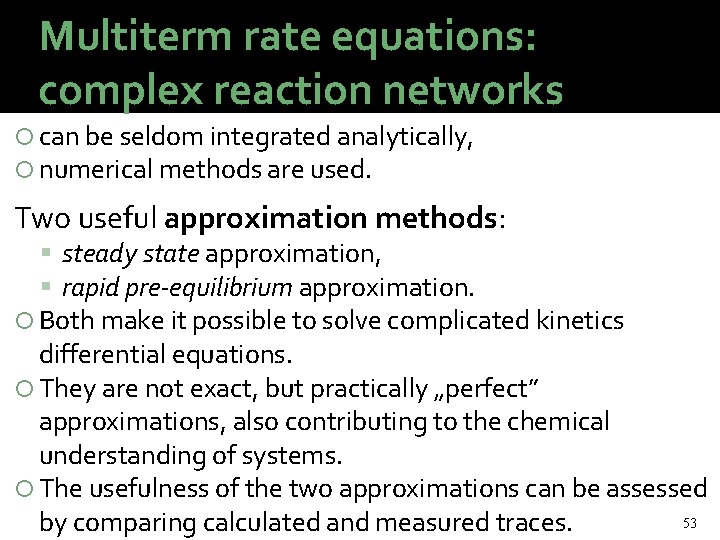
Multiterm rate equations: complex reaction networks can be seldom integrated analytically, numerical methods are used. Two useful approximation methods: steady state approximation, rapid pre-equilibrium approximation. Both make it possible to solve complicated kinetics differential equations. They are not exact, but practically „perfect” approximations, also contributing to the chemical understanding of systems. The usefulness of the two approximations can be assessed 53 by comparing calculated and measured traces.
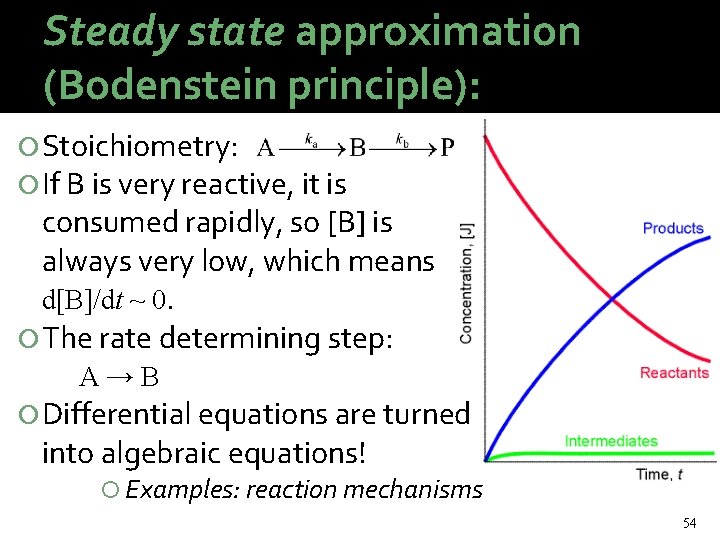
Steady state approximation (Bodenstein principle): Stoichiometry: If B is very reactive, it is consumed rapidly, so [B] is always very low, which means d[B]/dt ~ 0. The rate determining step: A→B Differential equations are turned into algebraic equations! Examples: reaction mechanisms 54
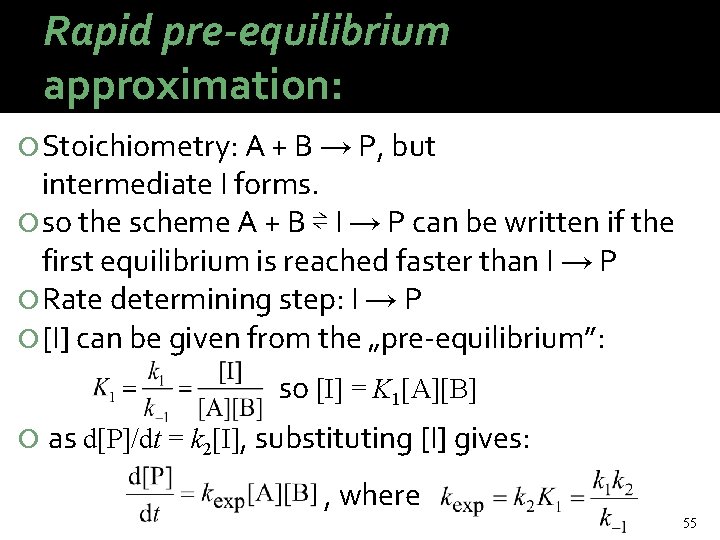
Rapid pre-equilibrium approximation: Stoichiometry: A + B → P, but intermediate I forms. so the scheme A + B ⇌ I → P can be written if the first equilibrium is reached faster than I → P Rate determining step: I → P [I] can be given from the „pre-equilibrium”: so [I] = K 1[A][B] as d[P]/dt = k 2[I], substituting [I] gives: , where 55
![Determining the rate equation Rate equation v dcdt kAαBβCγ 1 Determination of Determining the rate equation: Rate equation: v = dc/dt = k[A]α[B]β[C]γ 1. Determination of](https://slidetodoc.com/presentation_image_h2/81d4bb159f6fcac9231140af0e61230c/image-56.jpg)
Determining the rate equation: Rate equation: v = dc/dt = k[A]α[B]β[C]γ 1. Determination of all orders of reaction (α, β, γ, …) Summing the individual orders gives the net order of reaction. 2. Calculation of rate constant k 56
![Determining the rate equation Rate equation v dcdt kAαBβCγ 1 Determination of Determining the rate equation: Rate equation: v = dc/dt = k[A]α[B]β[C]γ 1. Determination of](https://slidetodoc.com/presentation_image_h2/81d4bb159f6fcac9231140af0e61230c/image-57.jpg)
Determining the rate equation: Rate equation: v = dc/dt = k[A]α[B]β[C]γ 1. Determination of all orders of reaction (α, β, γ, …) A. „trial-and-error” based on individual c – t traces. Plot the c – t pairs of points: ▪ if first order: lnc – t (semilogarithmic formula) ▪ if second order: 1/c – t (reciprocal formula) gives a straight line. ▪ Simple first and second order are common cases, but by no means exclusive, a „more certain” method is needed. 57
![Determining the rate equation Rate equation v dcdt kAαBβCγ 1 Determination of Determining the rate equation: Rate equation: v = dc/dt = k[A]α[B]β[C]γ 1. Determination of](https://slidetodoc.com/presentation_image_h2/81d4bb159f6fcac9231140af0e61230c/image-58.jpg)
Determining the rate equation: Rate equation: v = dc/dt = k[A]α[B]β[C]γ 1. Determination of all orders of reaction (α, β, γ, …) B. van’t Hoff method (initial rate method): ▪ Logarithm of the rate equation with initial values: lgv 0 = lgk + αlg[A]0 + βlg[B]0 + γlg[C]0 ▪ ▪ Set [B]0 and [C]0 so that [B] = [C] = constant (pseudozeroth order). Rate v 0 is measured at different [A]0, then the data pairs lgv 0 – lg[A]0 are plotted. 58
![Determining the rate equation Rate equation v dcdt kAαBβCγ 1 Determination of Determining the rate equation: Rate equation: v = dc/dt = k[A]α[B]β[C]γ 1. Determination of](https://slidetodoc.com/presentation_image_h2/81d4bb159f6fcac9231140af0e61230c/image-59.jpg)
Determining the rate equation: Rate equation: v = dc/dt = k[A]α[B]β[C]γ 1. Determination of all orders of reaction (α, β, γ, …) B. van’t Hoff method (initial rate method): ▪ ▪ Data pairs lgv 0 – lg[A]0 are plotted. The slope of the straight line is the α order of reaction with respect to reactant A. ▪ Then change [B]0 And keep [A]0 and [C]0 constant, so order β with respect to B is obtained. ▪ Initial values may be used, but rates at any other time may be useful for evaluation. 59
![Determining the rate equation Rate equation v dcdt kAαBβCγ 1 Determination of Determining the rate equation: Rate equation: v = dc/dt = k[A]α[B]β[C]γ 1. Determination of](https://slidetodoc.com/presentation_image_h2/81d4bb159f6fcac9231140af0e61230c/image-60.jpg)
Determining the rate equation: Rate equation: v = dc/dt = k[A]α[B]β[C]γ 1. Determination of all orders of reaction (α, β, γ, …) The individual orders of reactions may be: small positive integers (1, 2, 3) ▪ no explanation needed, a natural case small negative integers (-1, -2, -3) ▪ e. g. interpretation by rapid pre-equilibrium small positive/negative fractional numbers (1/2, 3/2) ▪ (e. g. parallel paths with different orders of reaction) ▪ e. g. dimer dissociation as a pre-equilibrium ▪ e. g. chain reactions 0 (this can be directly seen from the data, no plot needed) ▪ e. g. pre-equilibrium (iodination of acetone) ▪ e. g. certain surface reactions, drug intake processes 60
![Determining the rate equation Rate equation v dcdt kAαBβCγ 1 Determination of Determining the rate equation: Rate equation: v = dc/dt = k[A]α[B]β[C]γ 1. Determination of](https://slidetodoc.com/presentation_image_h2/81d4bb159f6fcac9231140af0e61230c/image-61.jpg)
Determining the rate equation: Rate equation: v = dc/dt = k[A]α[B]β[C]γ 1. Determination of all orders of reaction (α, β, γ, …) 2. Calculation of the rate constant k Numerical calculations from measured v rates (mol dm-3 s-1) and the known form of the rate equation. dimension of k: [k 1] = s-1, [k 2] = mol-1 dm 3 s-1 etc. Equilibrium constant K is the ratio of the rate constants k+ and k–– both numerically and dimensionally: K = k+/k – It is useful to compare these to other values of K, obtained from independent measurements. 61
Organic molecules vs inorganic molecules
States that atoms ions and molecules must collide to react
States of matter foldable
Four states of matter
Four states of matter
5 states of matter
States of matter thermal energy
Changing states of matter
Phet states of matter basics
5 states of matter
Diagram of the states of matter
The kinetic theory of matter states that
Slave states free states
Northern and southern states
Classification of matter section 1 composition of matter
Chapter 12 states of matter
Chapter 10 review states of matter section 4
States of matter basics
States of matter foldable
Heat thermal energy and temperature
Uses of heat
The fundamental difference between states of matter is the
Stayes of matter
Plasma particles arrangement
Function of grey matter and white matter
Section 1 composition of matter
Matter and energy concept map
Classification of matter concept map
Whats states of matter
States of matter
Properties of matter jeopardy
Chapter 2 section 1 classifying matter answer key
Label the cranial dura septa and associated sinuses.
Section 1 composition of matter chapter 15 answer key
Gray matter and white matter
Particle theory of matter examples
All matter is in constant motion
4 phases of matter
States of matter solid liquid gas
Interconversion of states of matter
Flow chart for classifying matter
Five states of matter
States of matter objectives
Ncl. caudatus
States of matter
Solid to gas
Example of deposition
States of matter jeopardy
5 states of matter
Flow of energy vs flow of matter
Jeopardy states of matter
Big states vs small states guard against tyranny
Chapter 11 - states of matter: liquids and solids
Curvilinear motion matter
Newton's laws vocabulary
Active rom
Periodic motion formula
An object in motion stays in motion
Chapter 2 motion section 1 describing motion answer key
Chapter 2 motion section 1 describing motion answer key
Section 1 describing motion
Chapter 11 section 1 measuring motion answer key