It takes glue to study the Glue Zisis
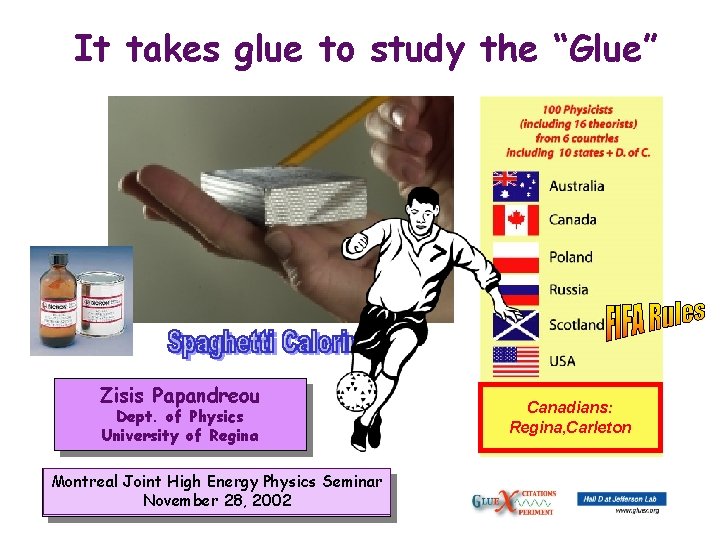
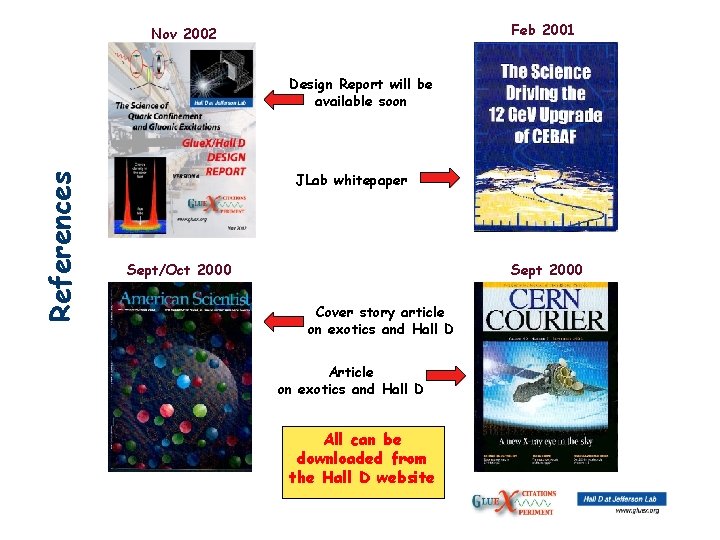
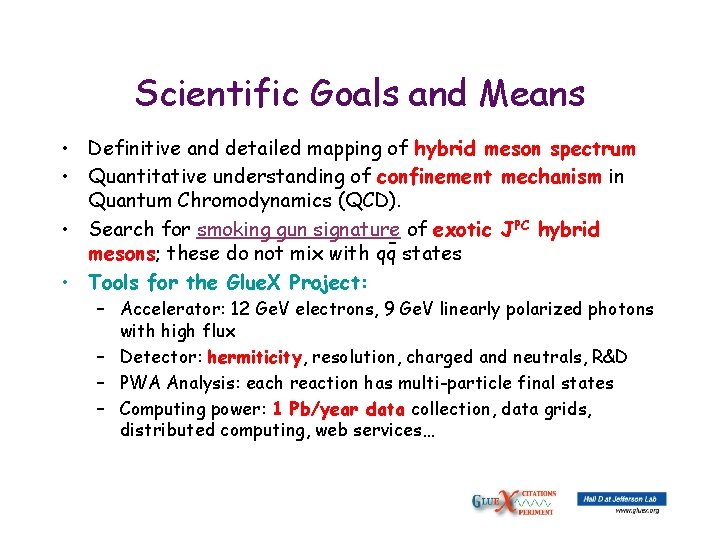
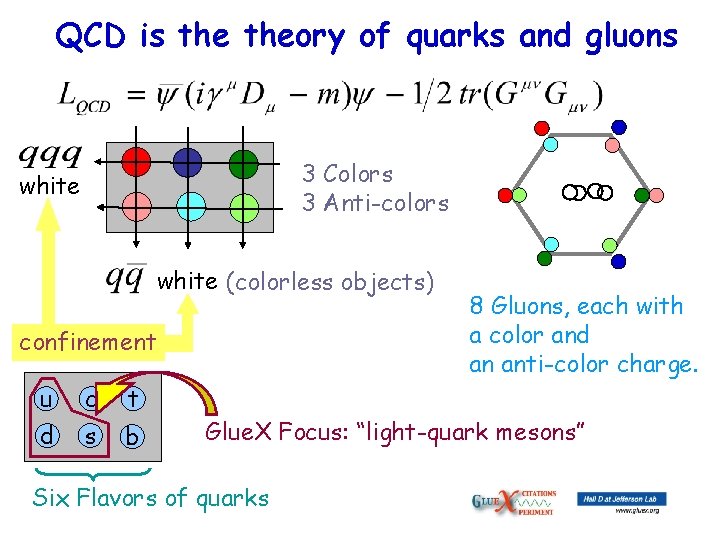
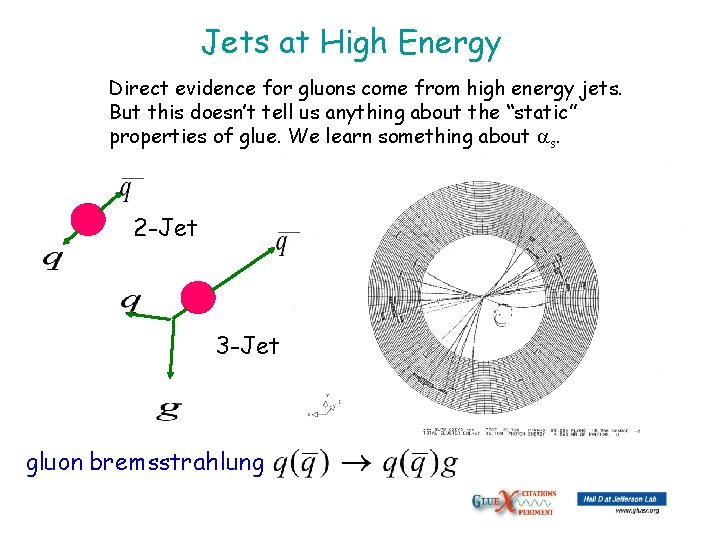
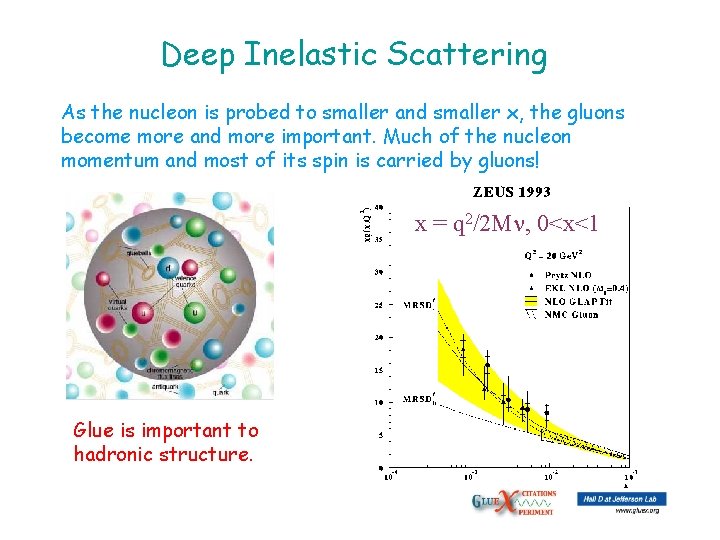
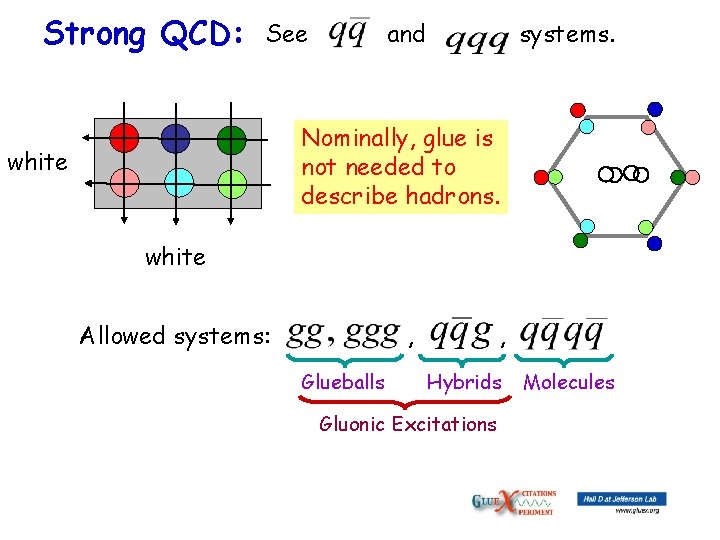
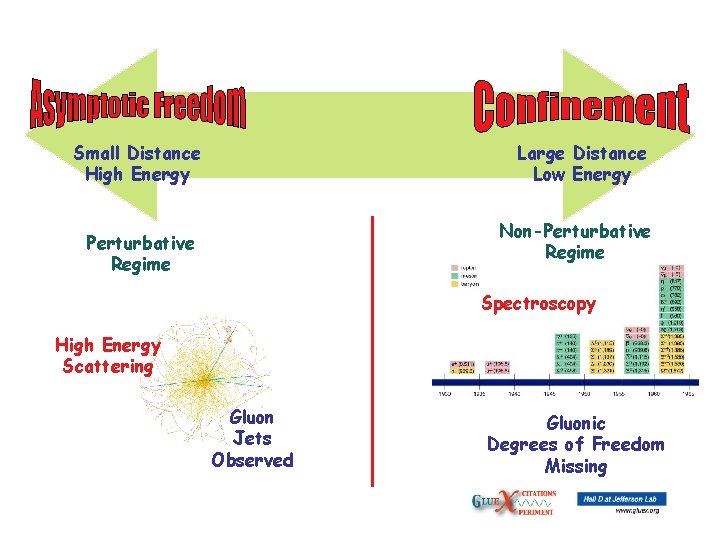
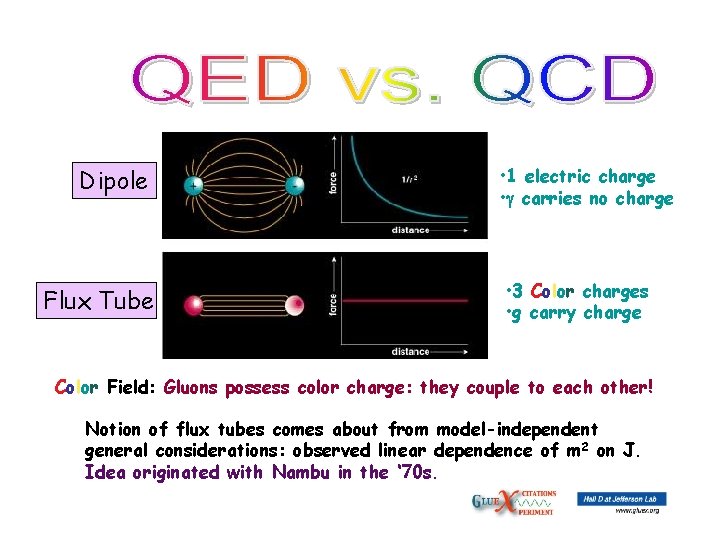
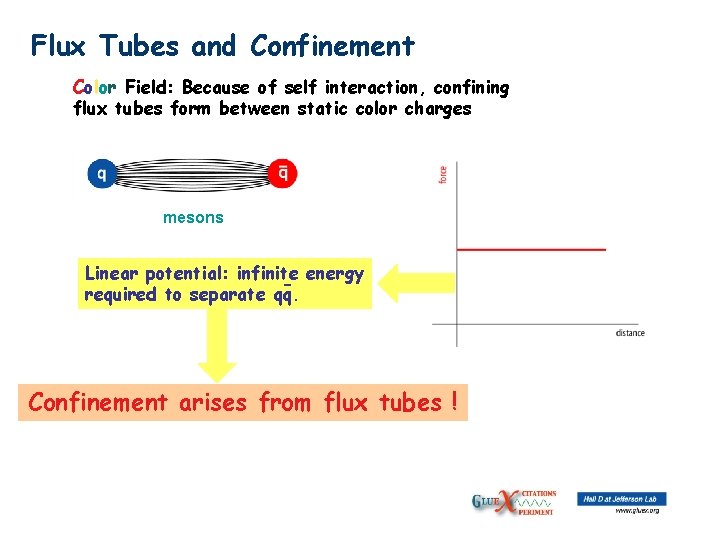
![Lattice QCD Flux tubes realized From G. Bali Vo( r) [Ge. V] 2. 0 Lattice QCD Flux tubes realized From G. Bali Vo( r) [Ge. V] 2. 0](https://slidetodoc.com/presentation_image_h2/7efb86a43acaf481f2d4d998a3fa7f22/image-11.jpg)
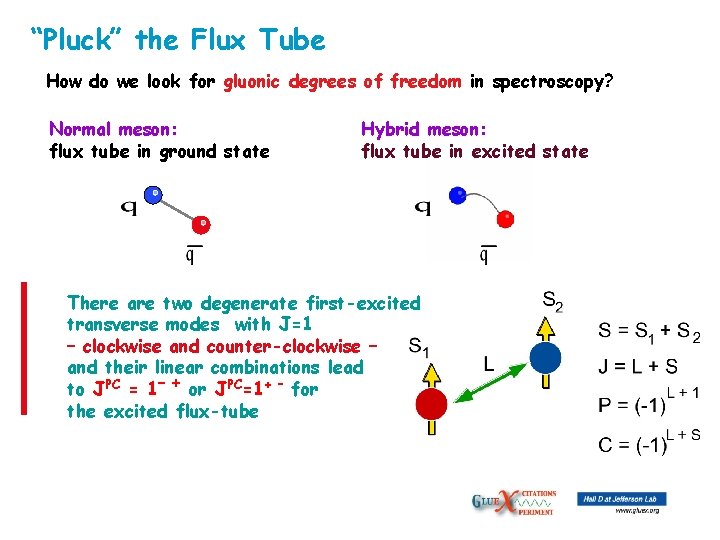
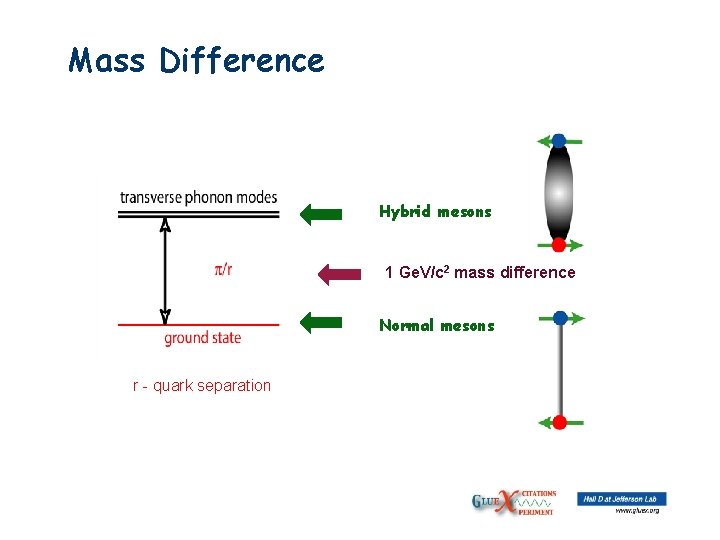
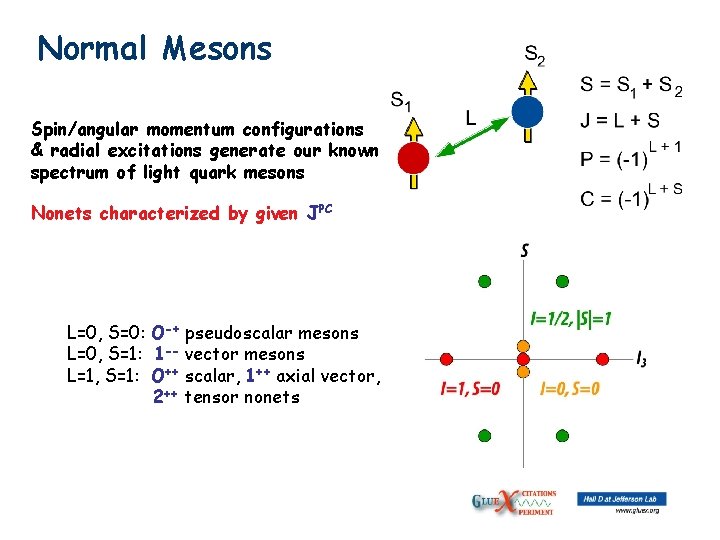
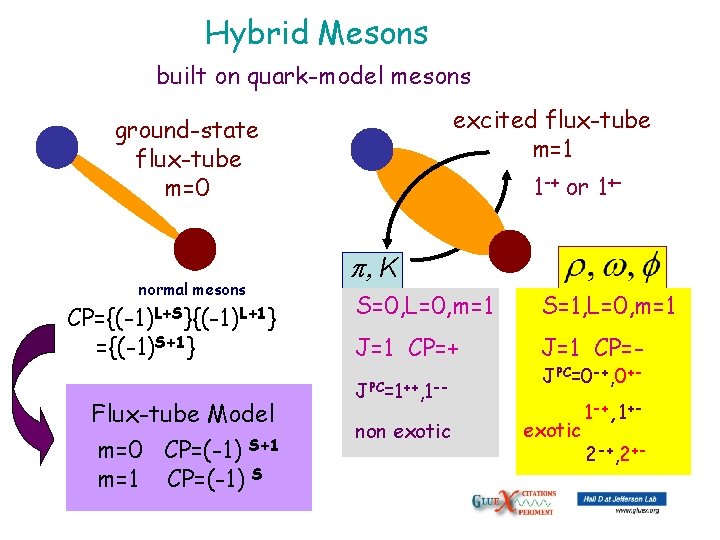
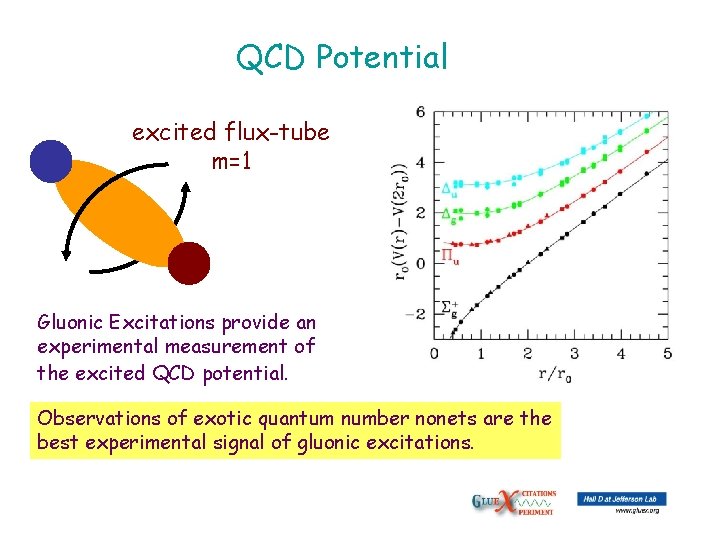
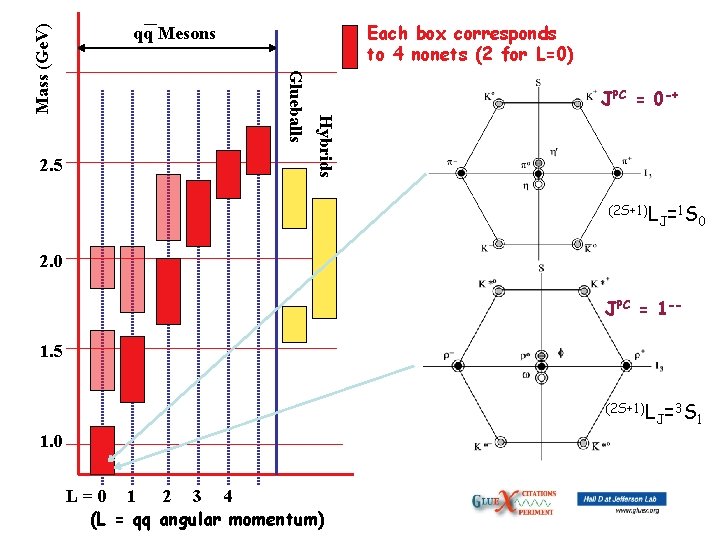
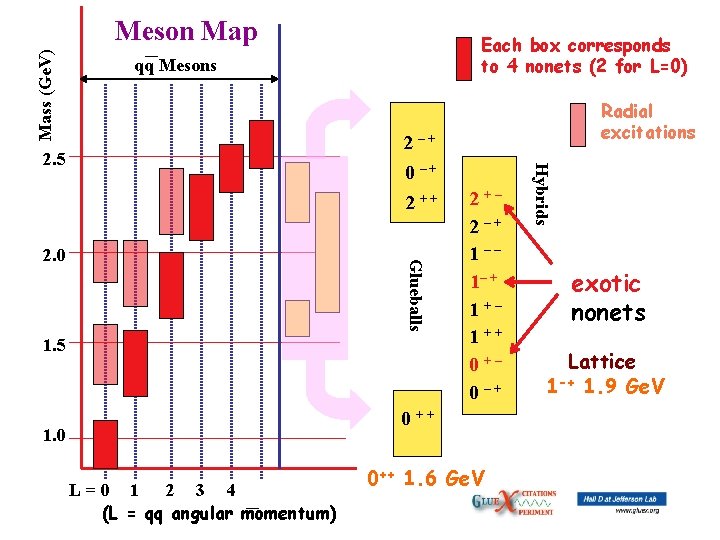
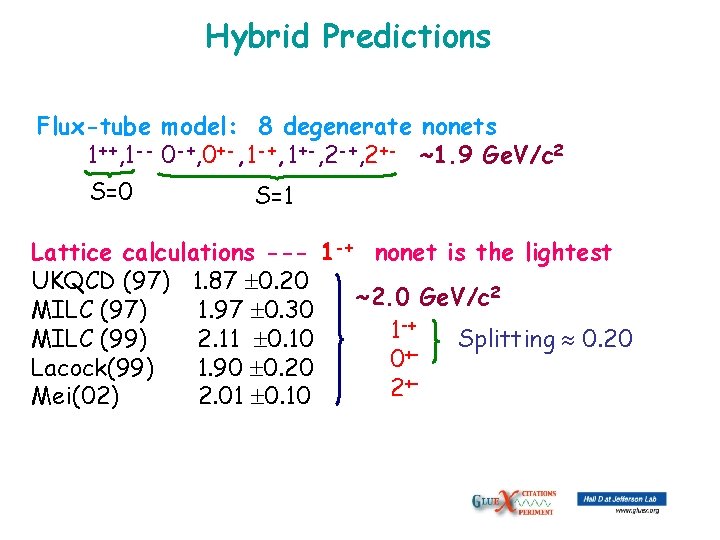
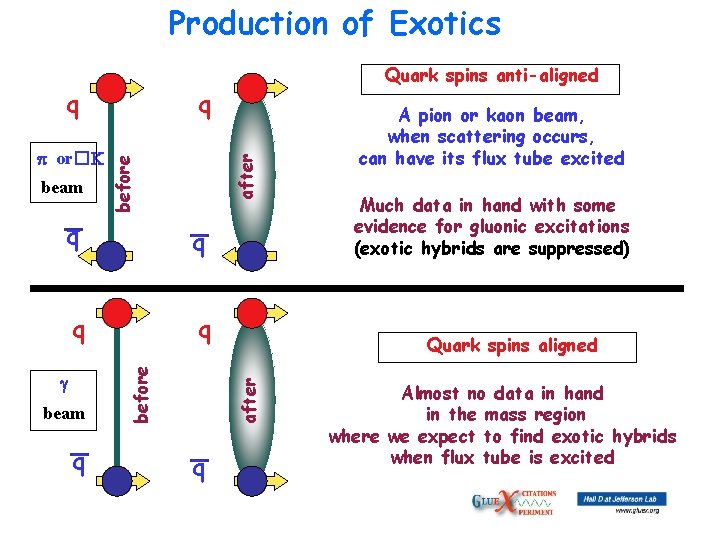
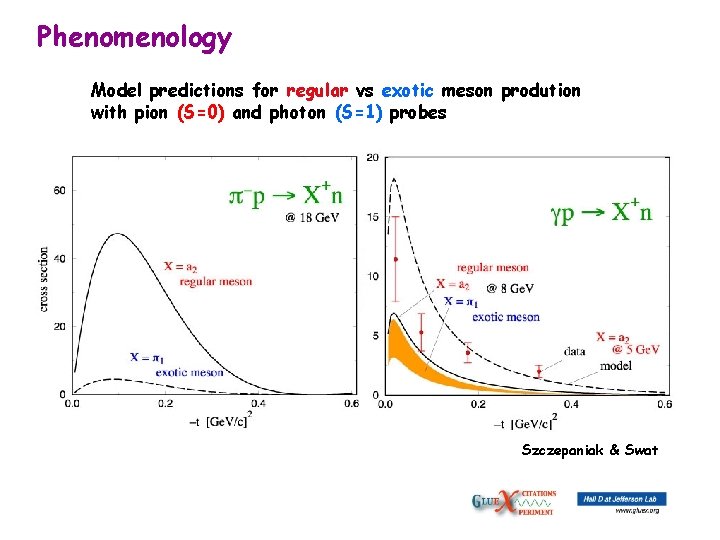
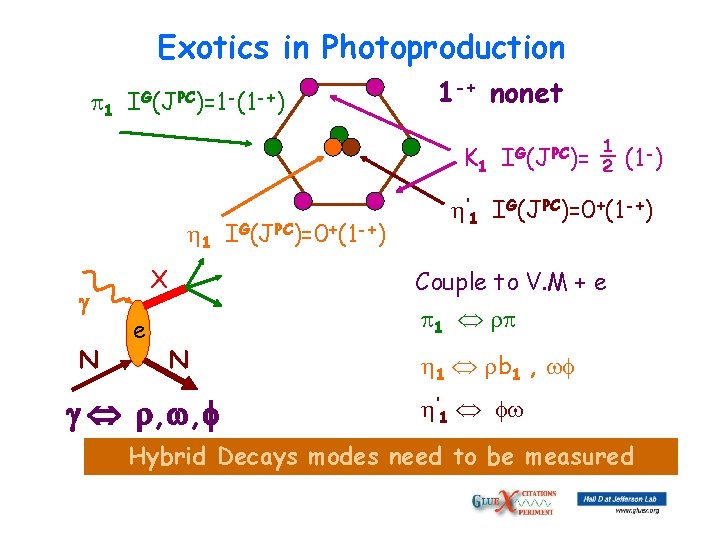
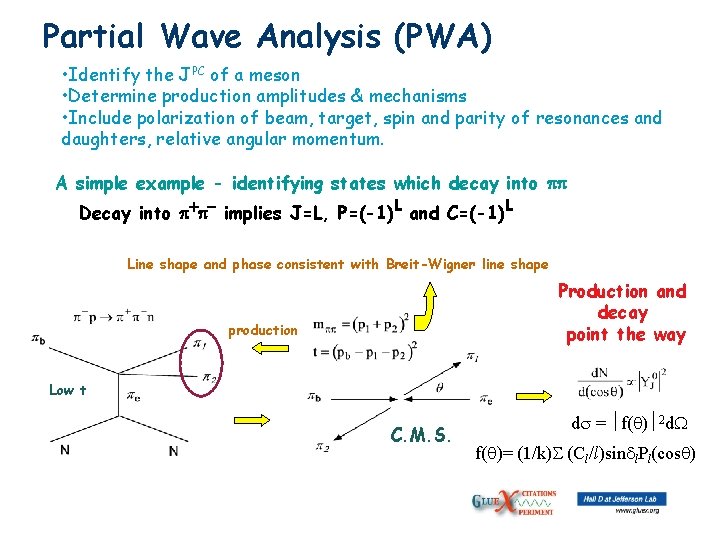
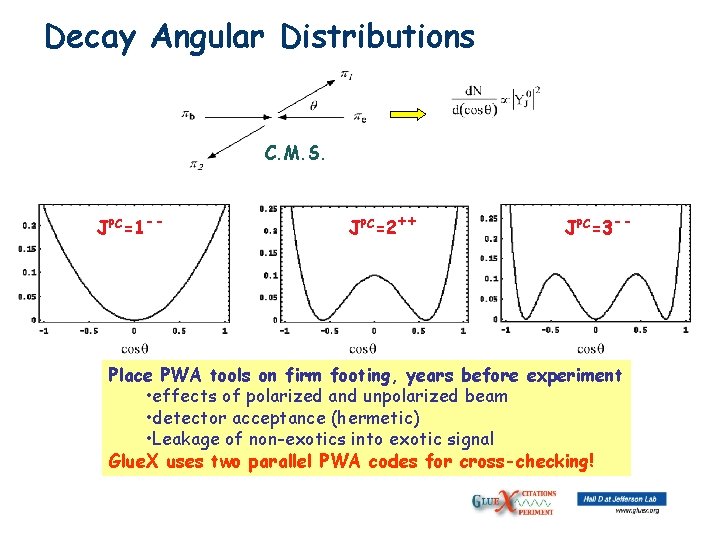
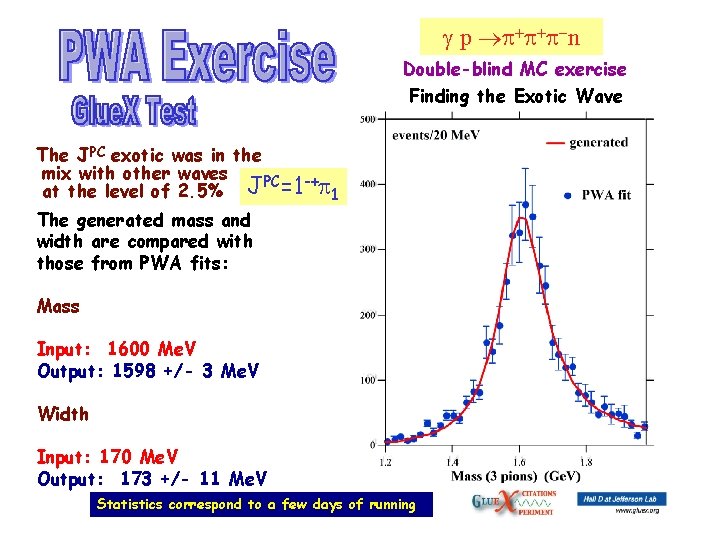
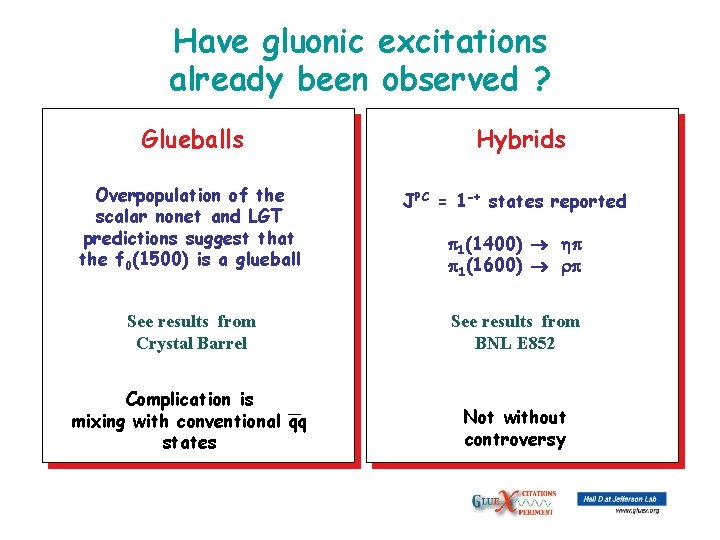
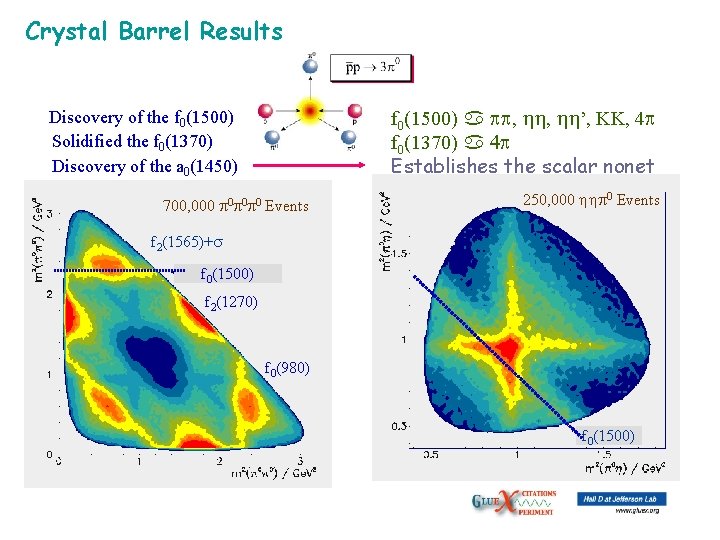
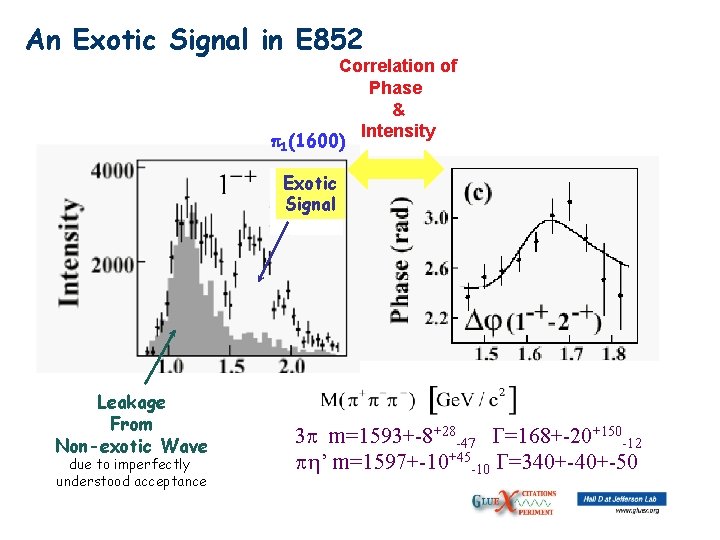
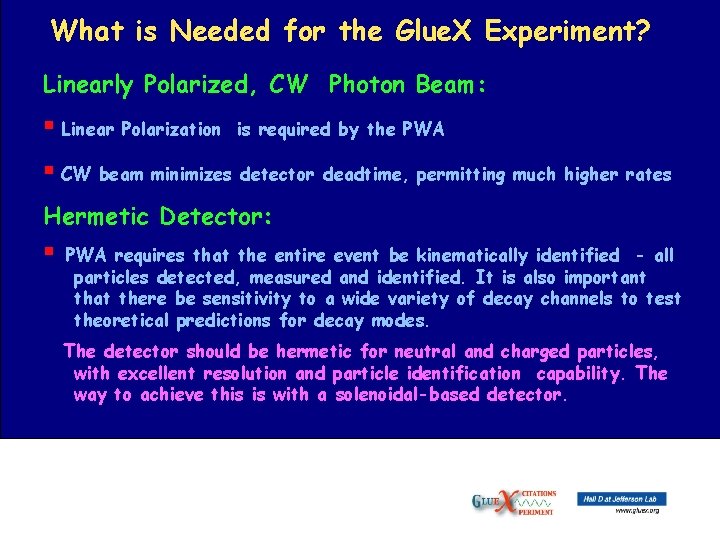
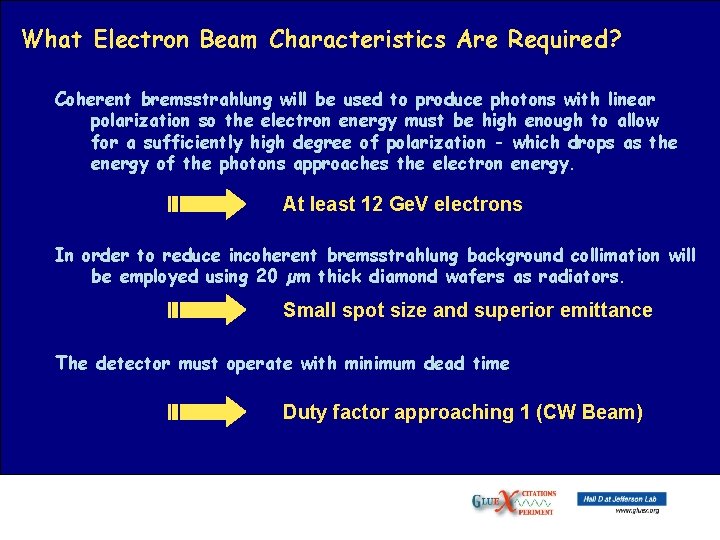
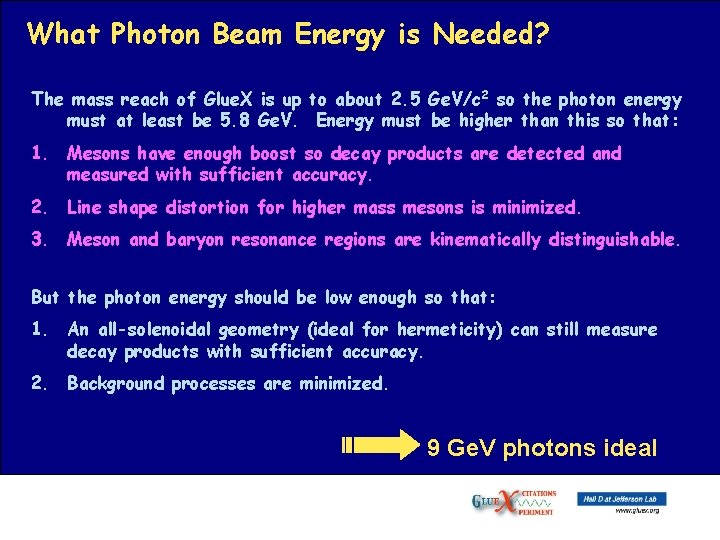
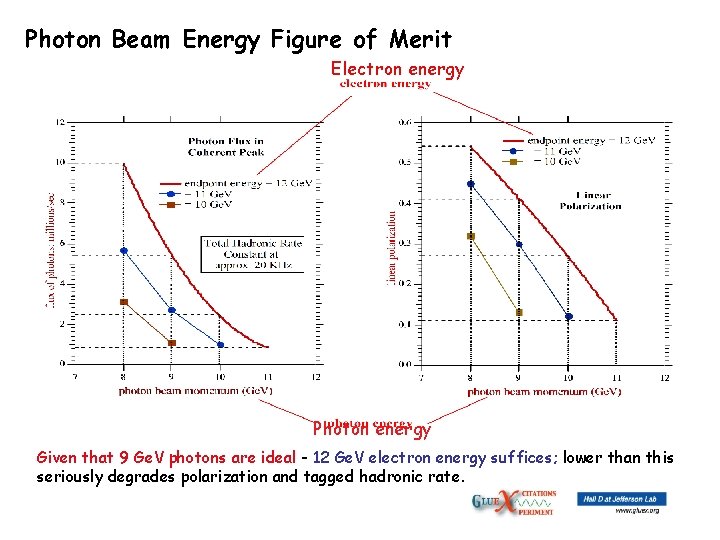
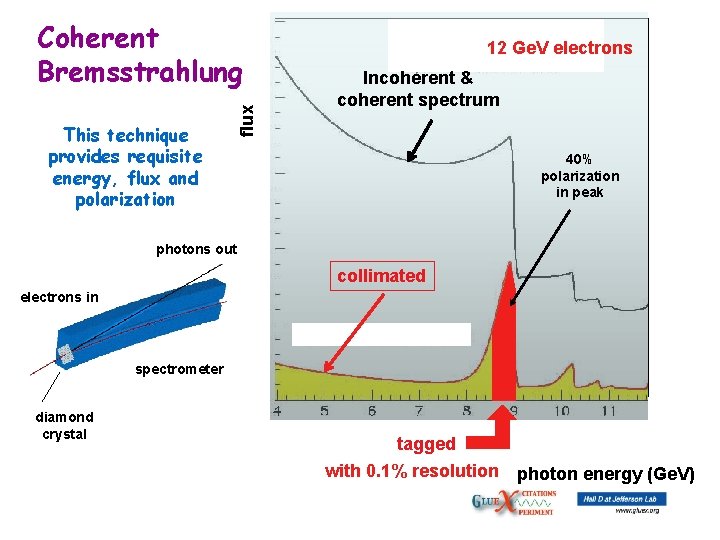
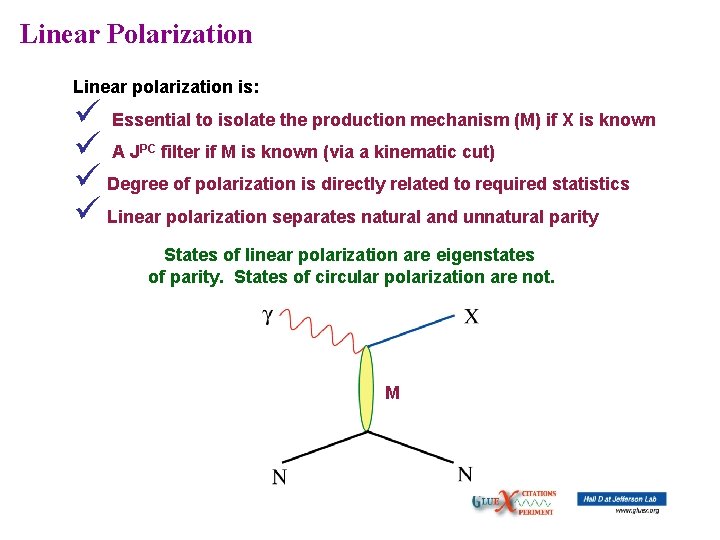
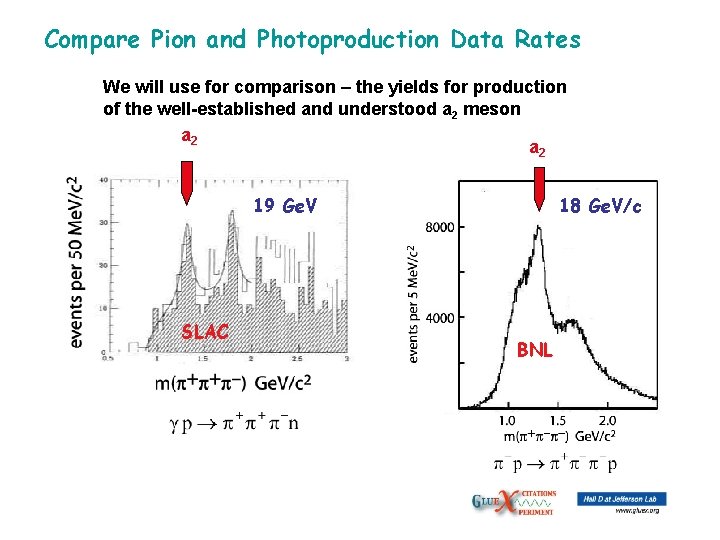
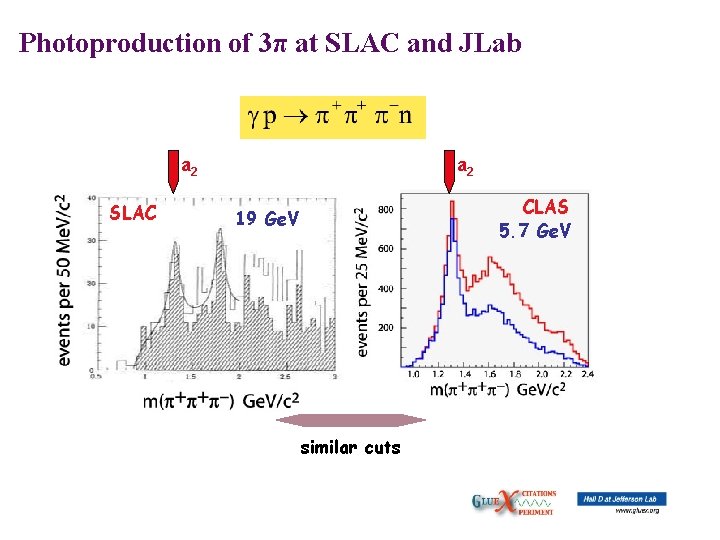
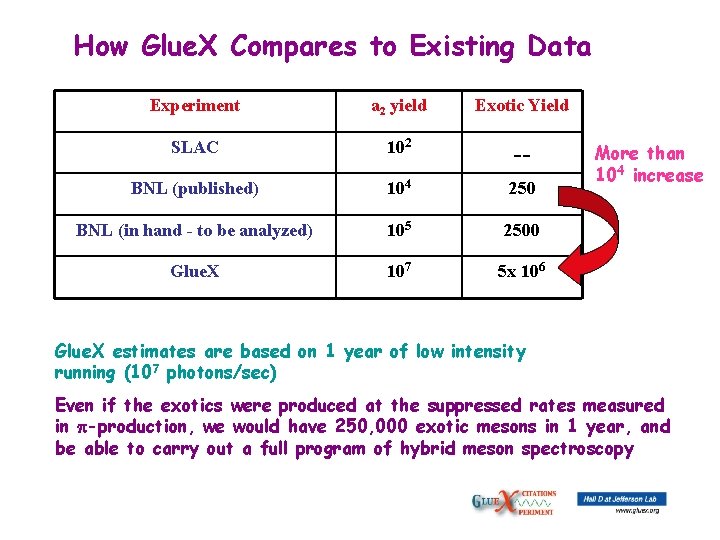
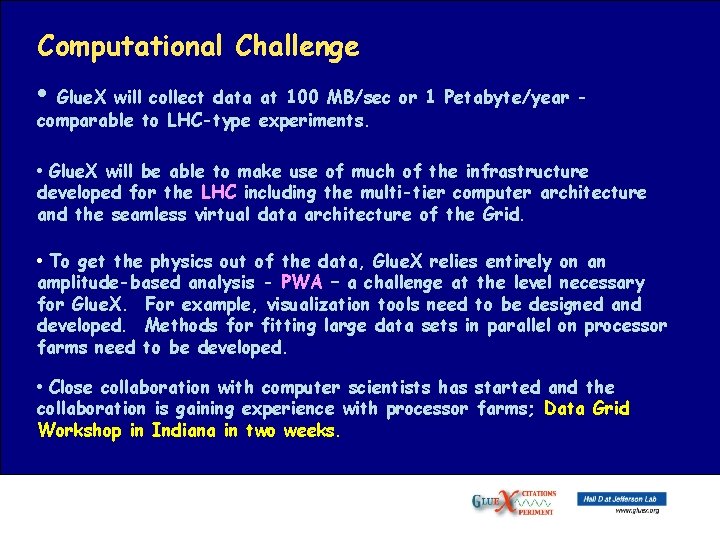
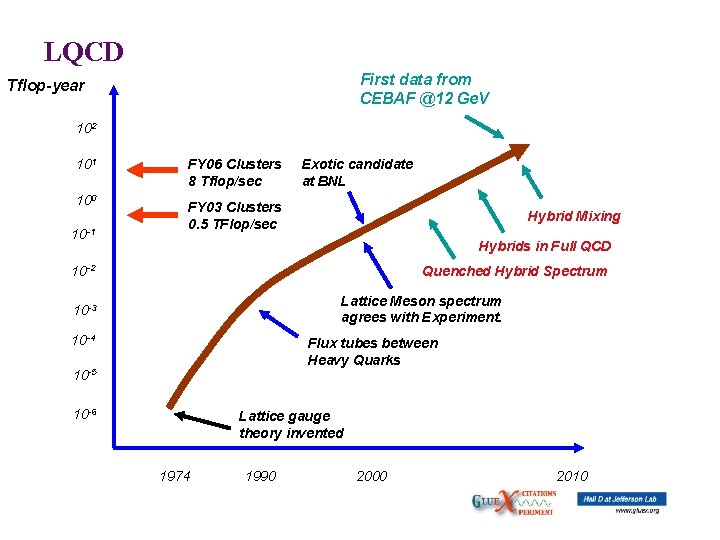
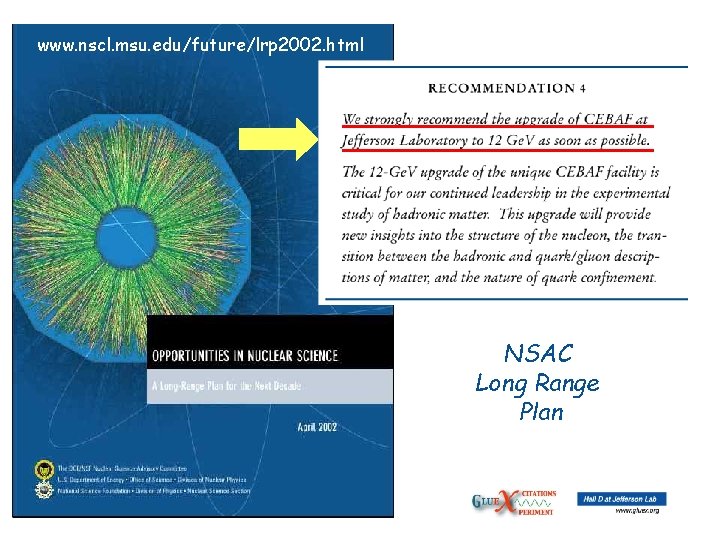
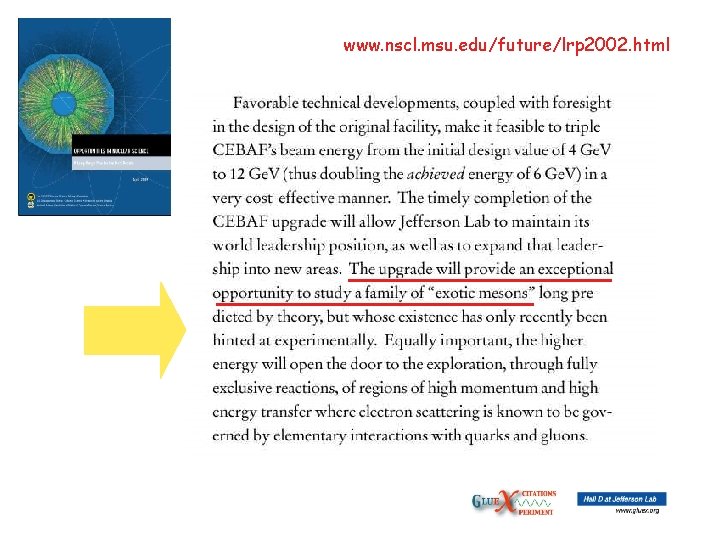
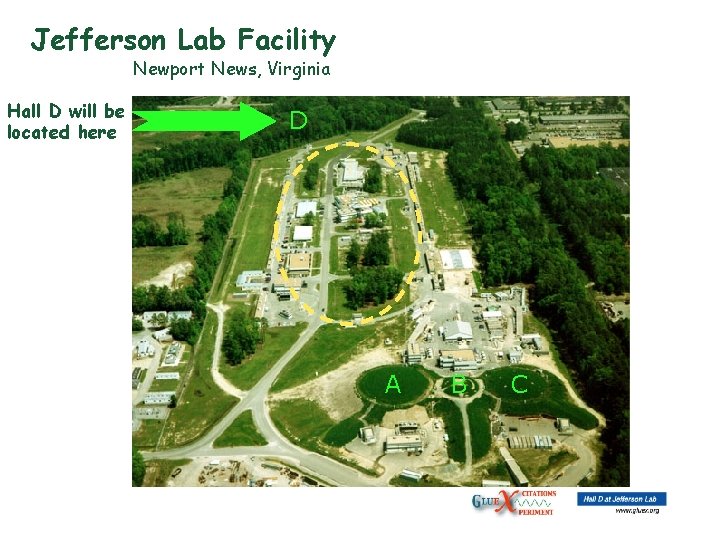
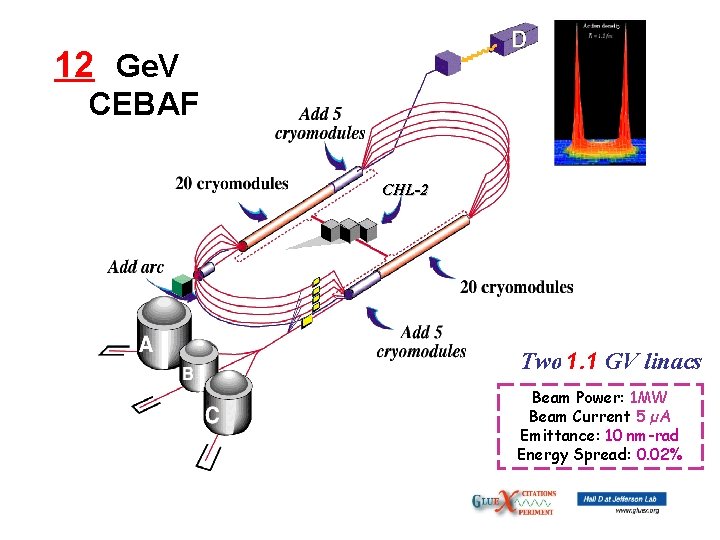
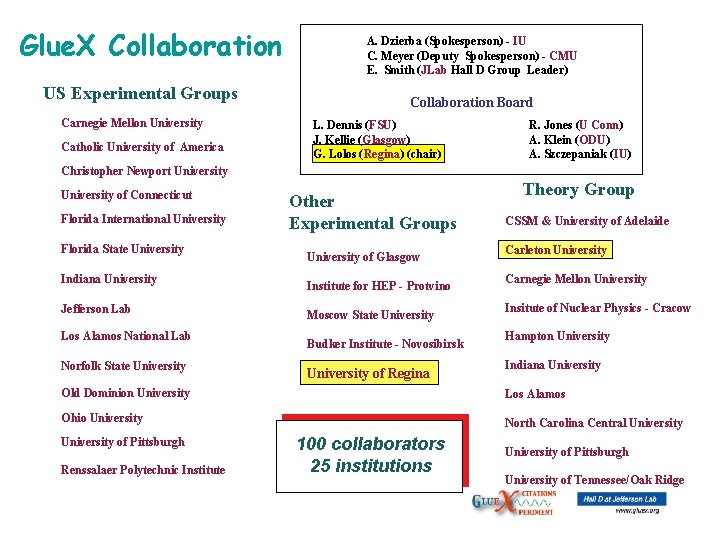
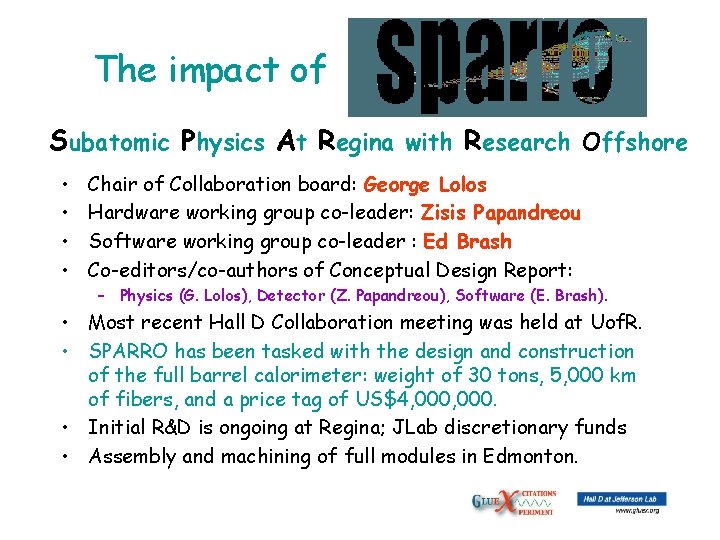
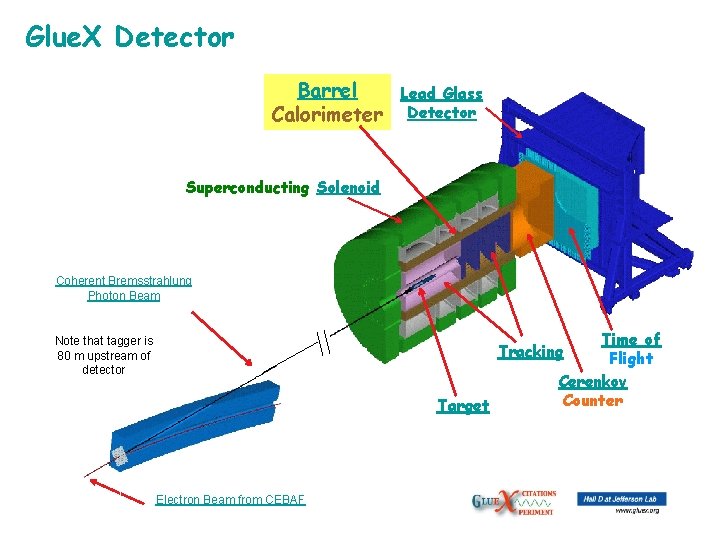
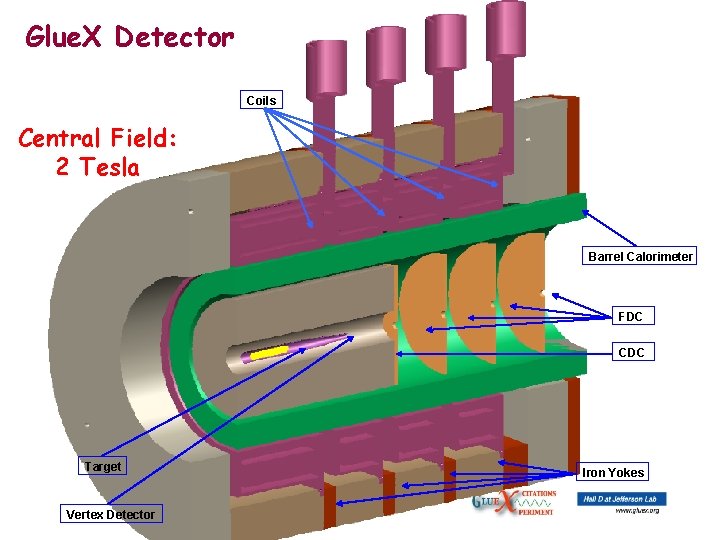
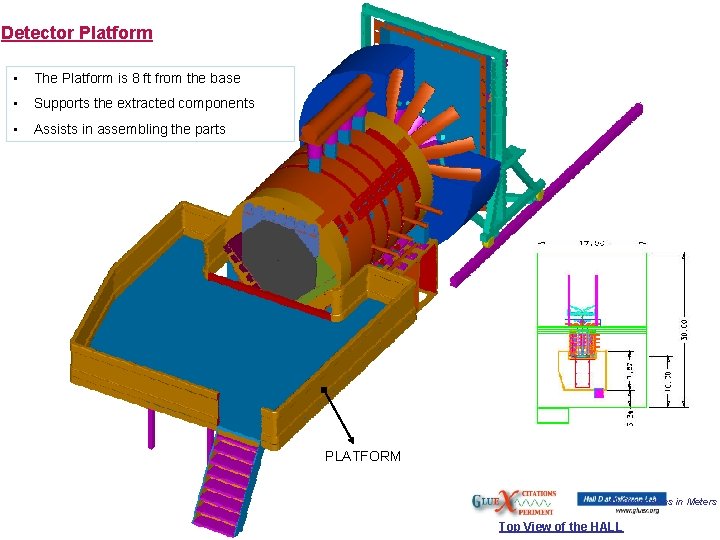
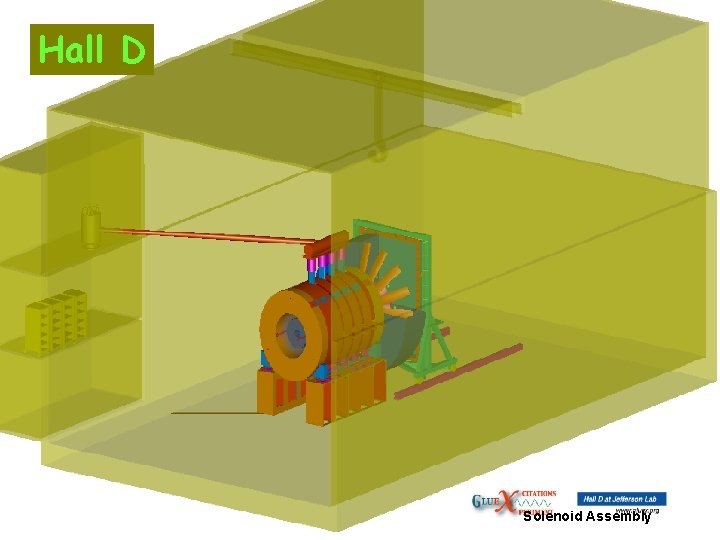
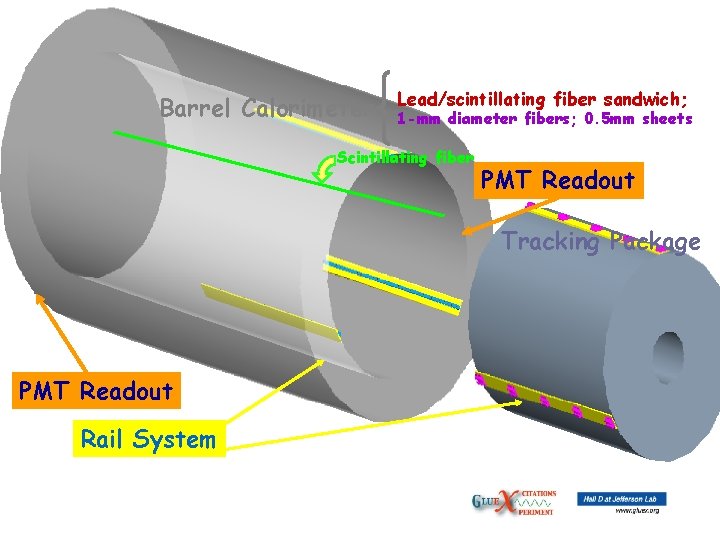
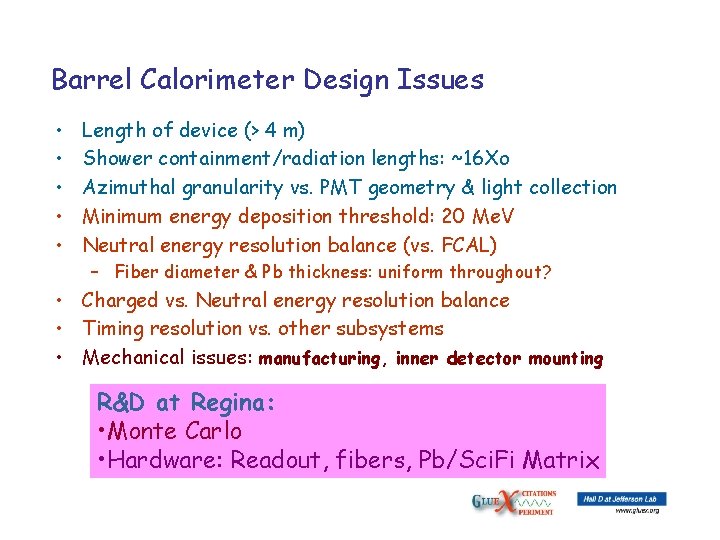
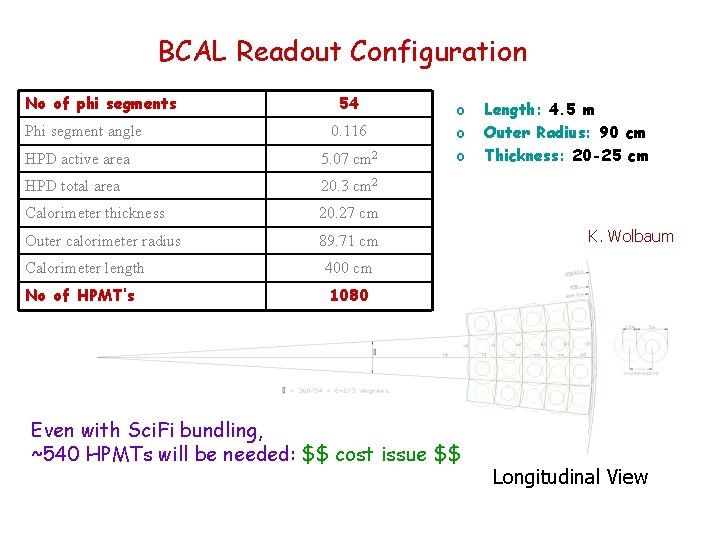
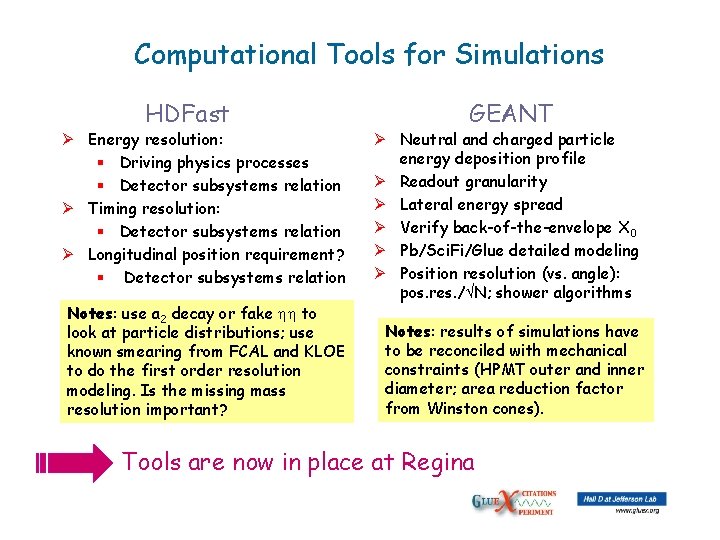
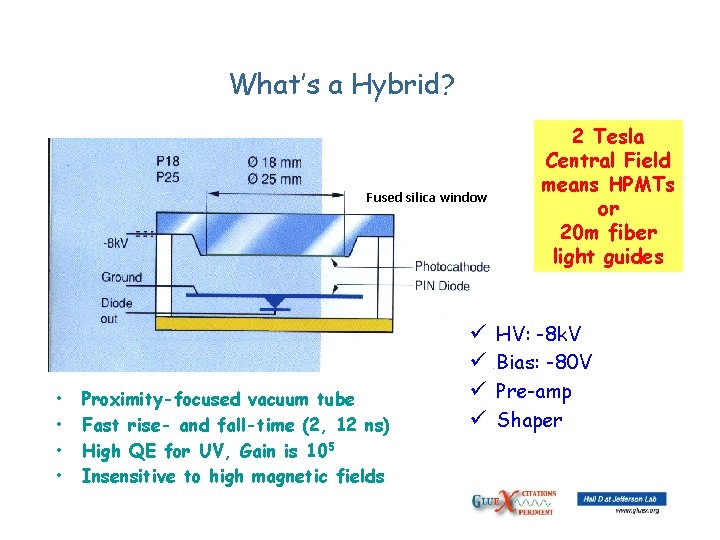
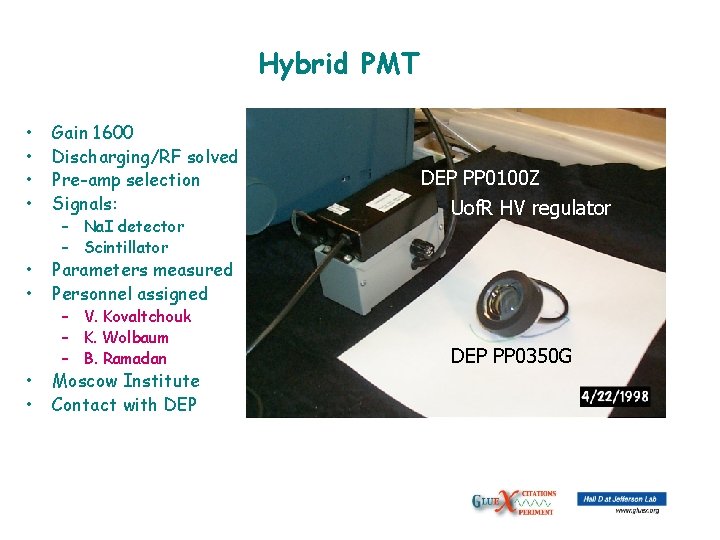
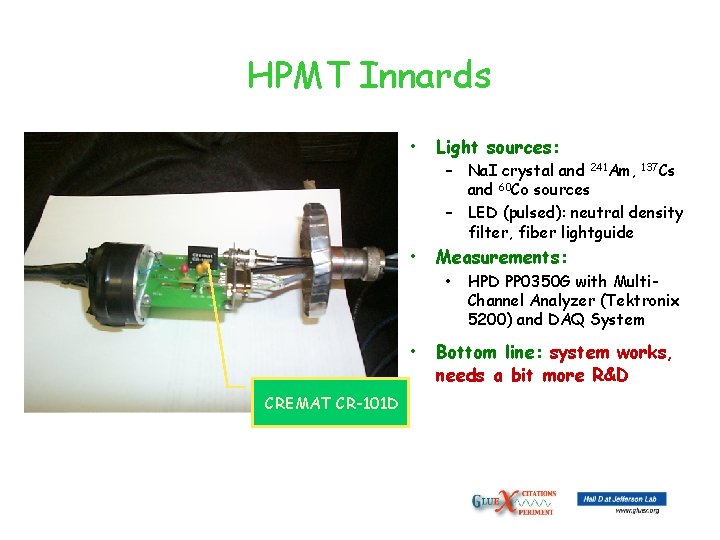
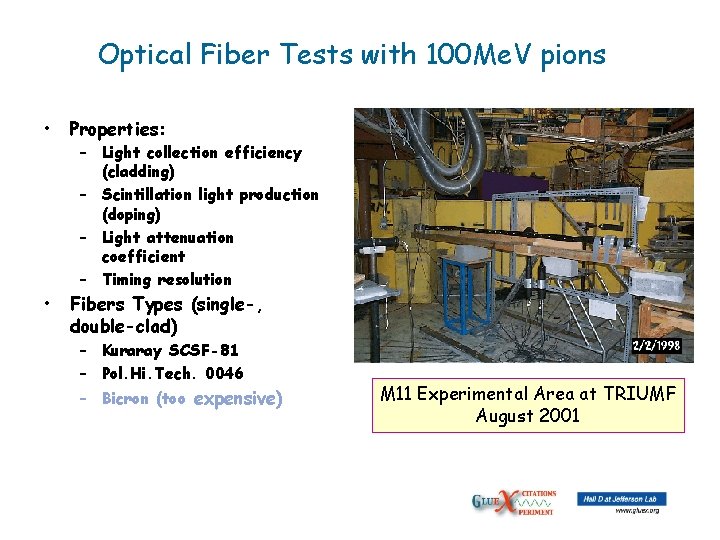
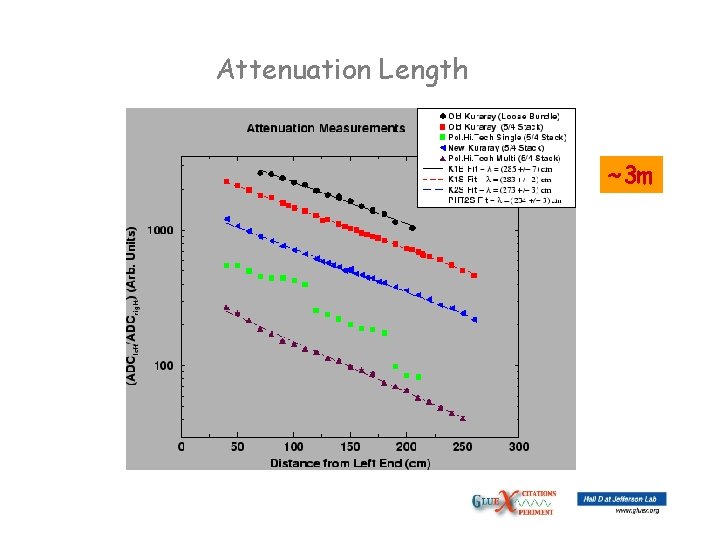
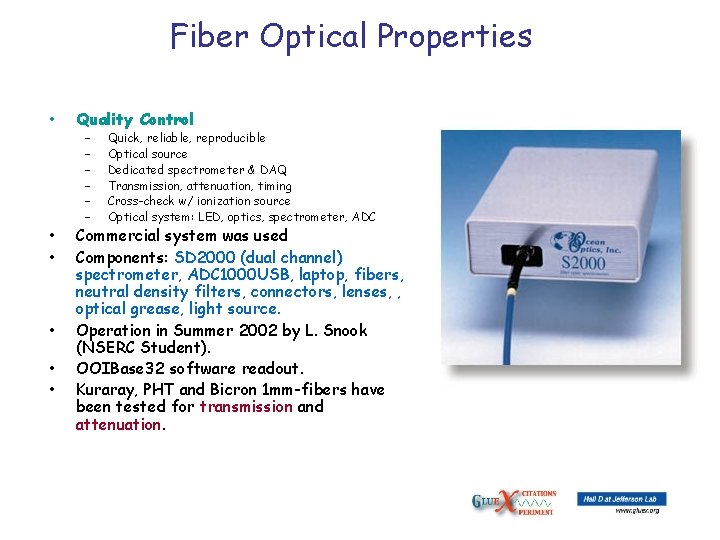
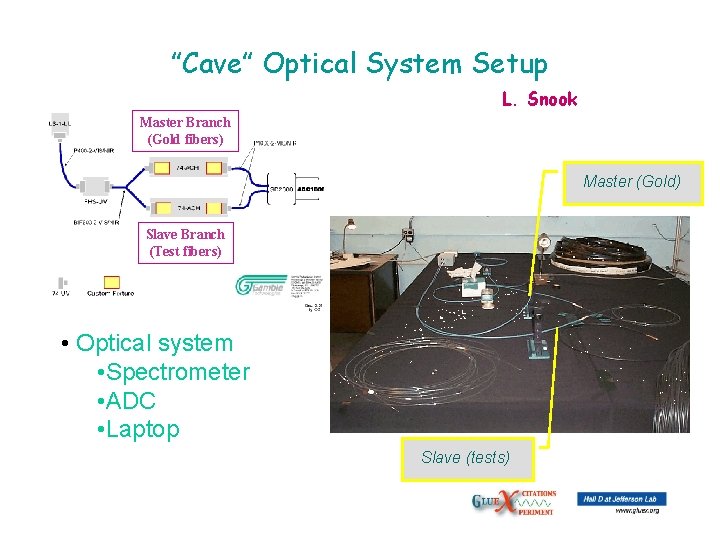
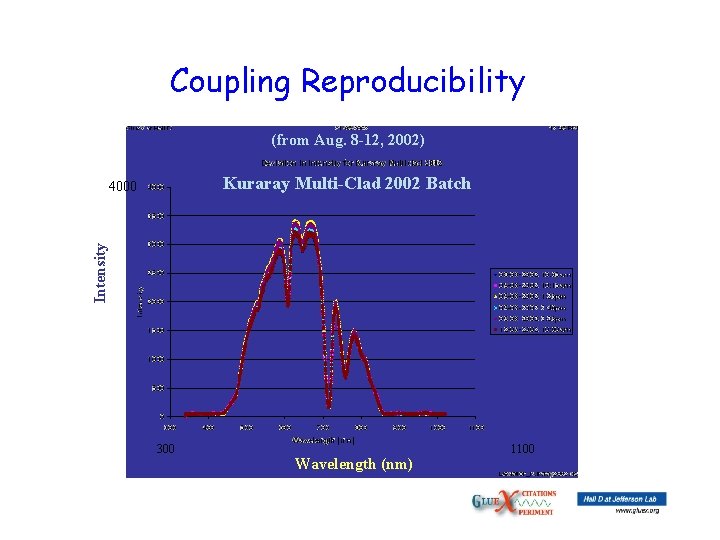
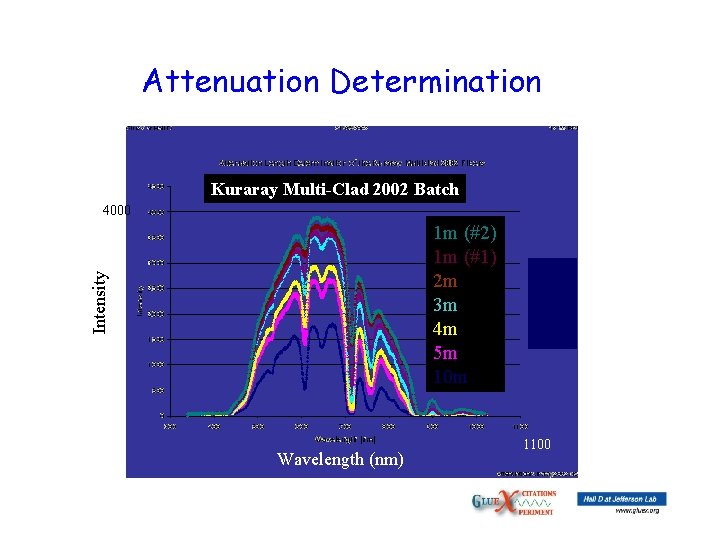
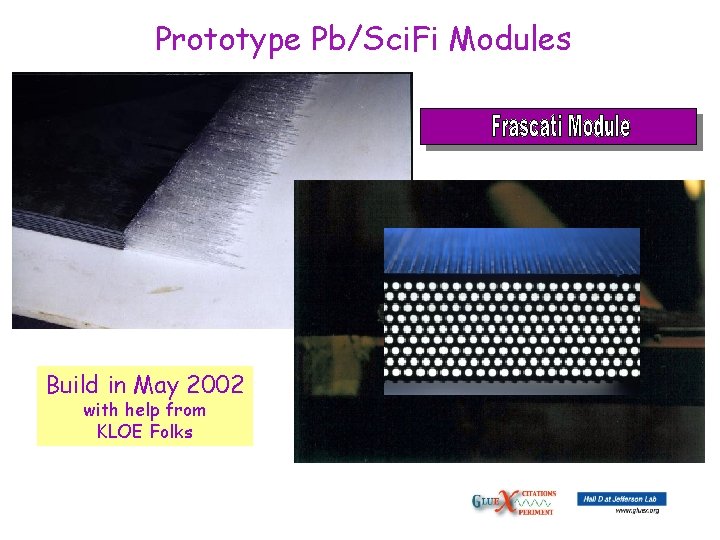
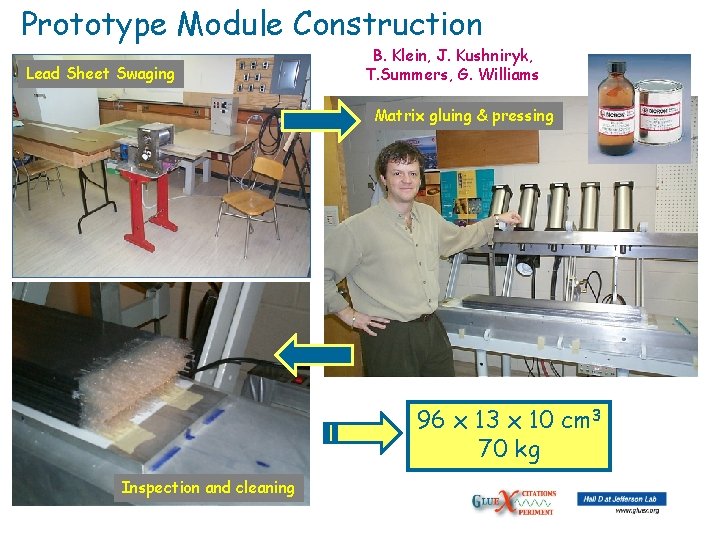
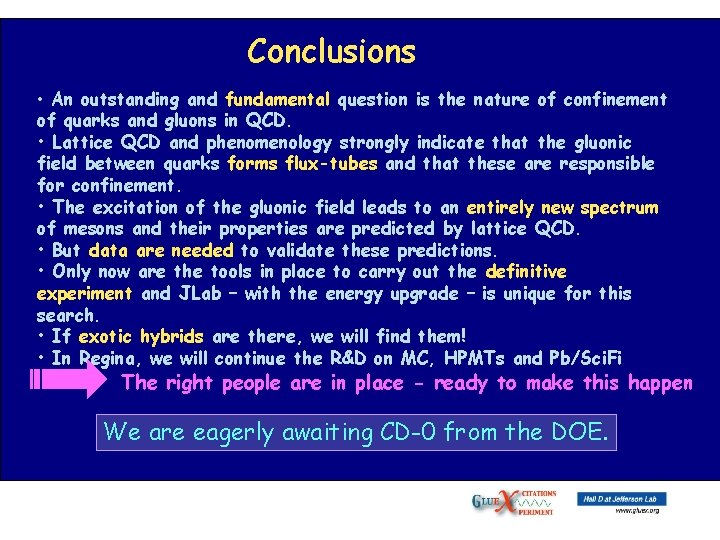
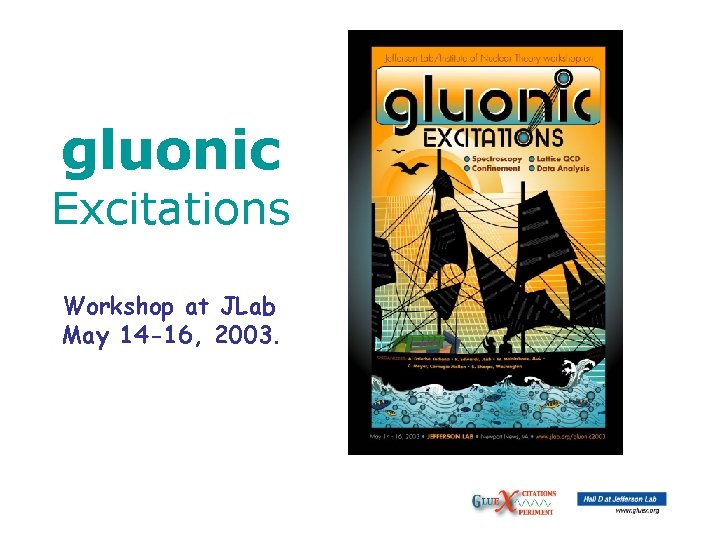
- Slides: 66
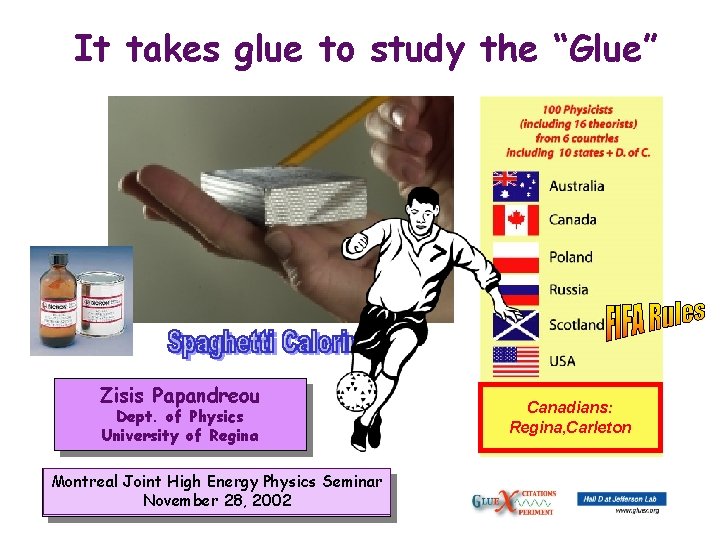
It takes glue to study the “Glue” Zisis Papandreou Dept. of Physics University of Regina Montreal Joint High Energy Physics Seminar November 28, 2002 Canadians: Regina, Carleton
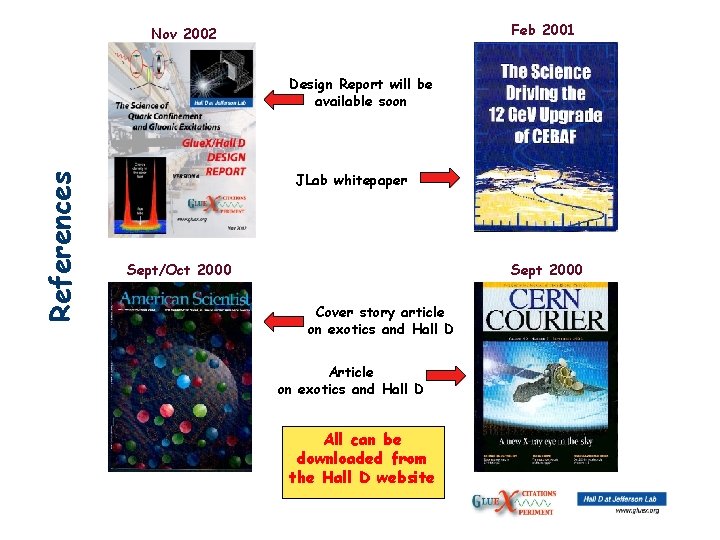
Feb 2001 Nov 2002 References Design Report will be available soon JLab whitepaper Sept/Oct 2000 Sept 2000 Cover story article on exotics and Hall D All can be downloaded from the Hall D website
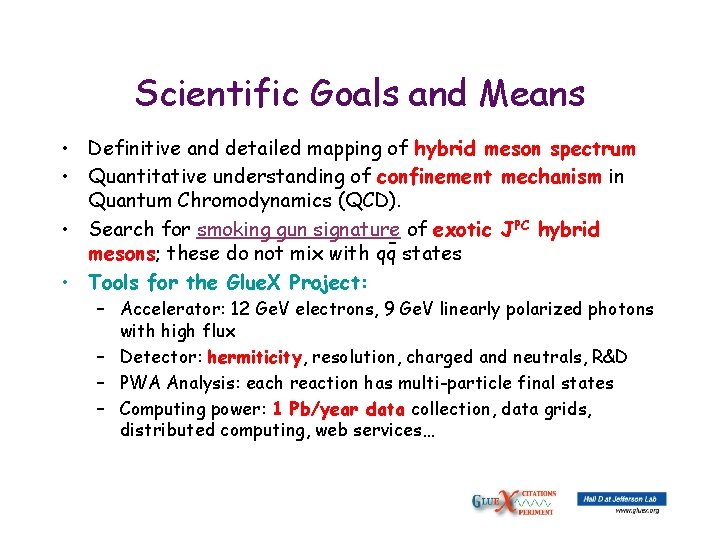
Scientific Goals and Means • Definitive and detailed mapping of hybrid meson spectrum • Quantitative understanding of confinement mechanism in Quantum Chromodynamics (QCD). • Search for smoking gun signature of exotic JPC hybrid mesons; these do not mix with qq states • Tools for the Glue. X Project: – Accelerator: 12 Ge. V electrons, 9 Ge. V linearly polarized photons with high flux – Detector: hermiticity, resolution, charged and neutrals, R&D – PWA Analysis: each reaction has multi-particle final states – Computing power: 1 Pb/year data collection, data grids, distributed computing, web services…
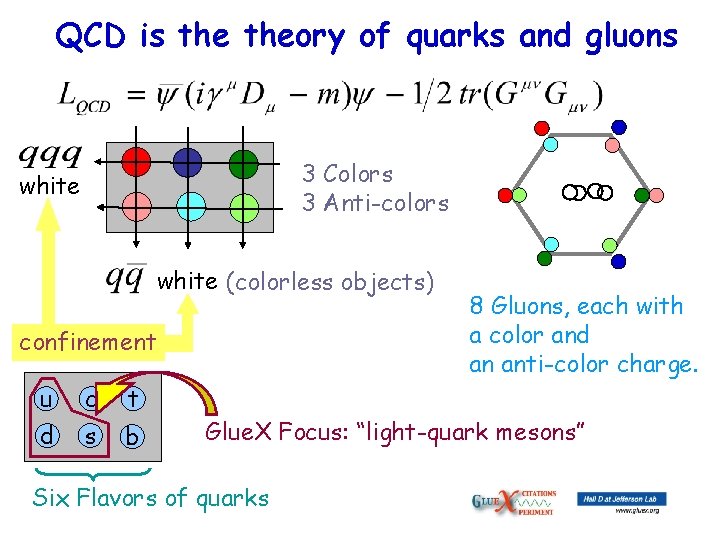
QCD is theory of quarks and gluons 3 Colors 3 Anti-colors white (colorless objects) confinement u c t d s b 8 Gluons, each with a color and an anti-color charge. Glue. X Focus: “light-quark mesons” Six Flavors of quarks
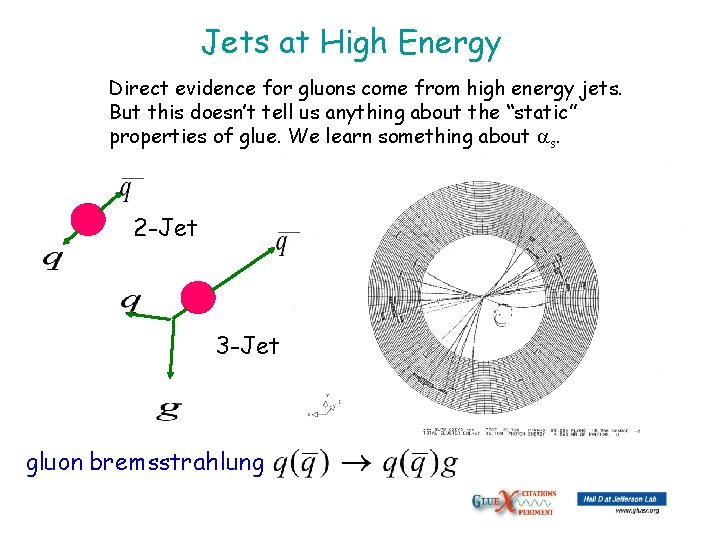
Jets at High Energy Direct evidence for gluons come from high energy jets. But this doesn’t tell us anything about the “static” properties of glue. We learn something about s. 2 -Jet 3 -Jet gluon bremsstrahlung
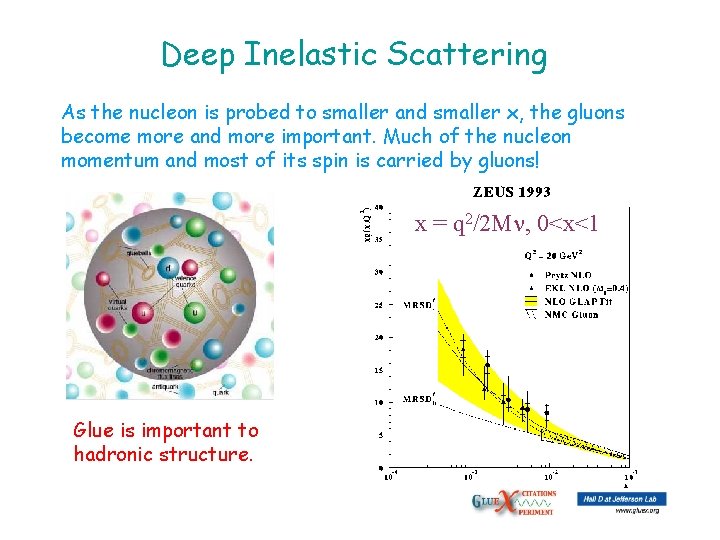
Deep Inelastic Scattering As the nucleon is probed to smaller and smaller x, the gluons become more and more important. Much of the nucleon momentum and most of its spin is carried by gluons! x = q 2/2 M , 0<x<1 Glue is important to hadronic structure.
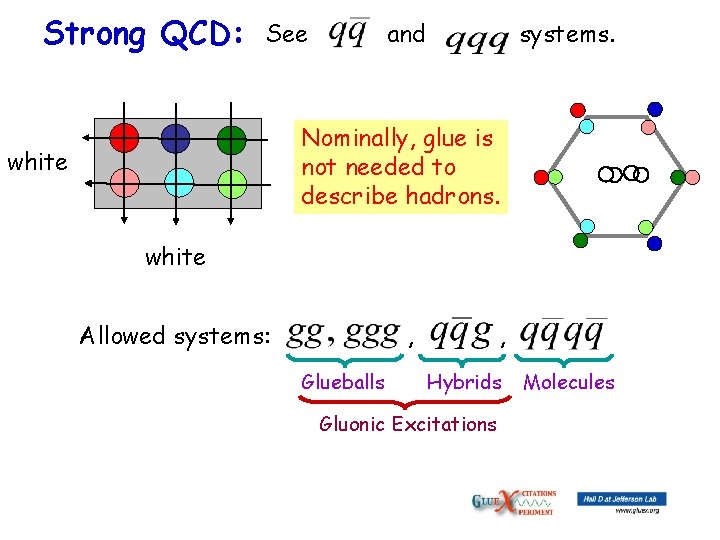
Strong QCD: See and systems. Nominally, glue is not needed to describe hadrons. white Allowed systems: , Glueballs , Hybrids Gluonic Excitations Molecules
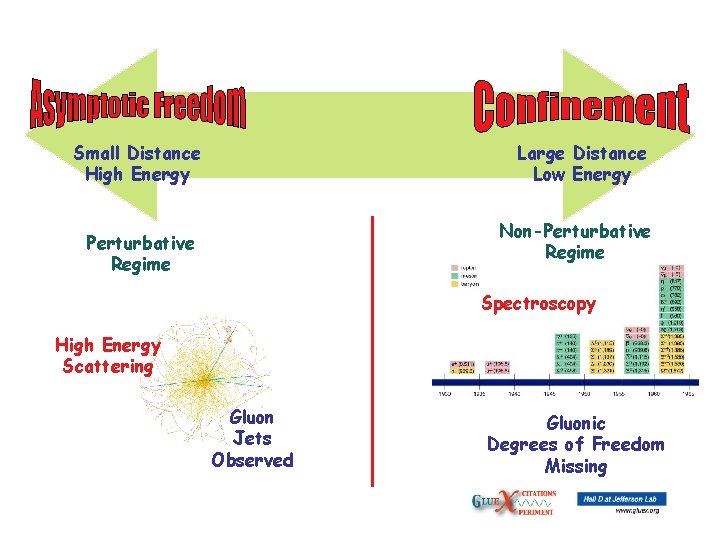
QCD and confinement Small Distance High Energy Large Distance Low Energy Non-Perturbative Regime Spectroscopy High Energy Scattering Gluon Jets Observed Gluonic Degrees of Freedom Missing
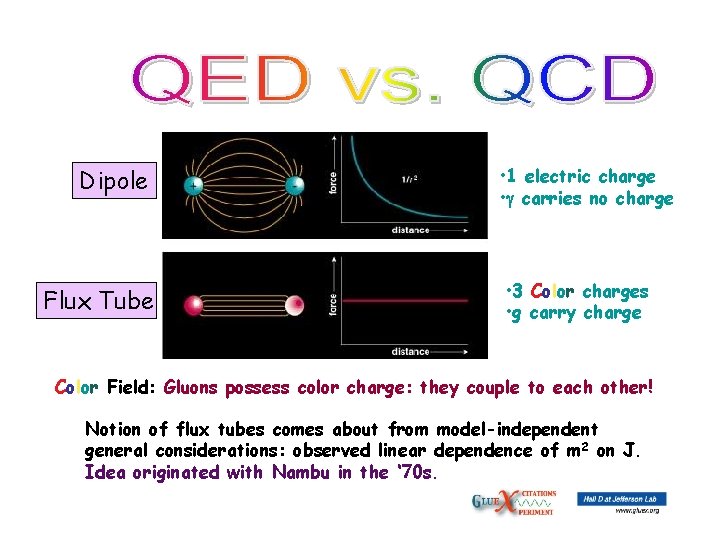
Flux Tubes Dipole Flux Tube • 1 electric charge • carries no charge • 3 Color charges • g carry charge Color Field: Gluons possess color charge: they couple to each other! Notion of flux tubes comes about from model-independent general considerations: observed linear dependence of m 2 on J. Idea originated with Nambu in the ‘ 70 s.
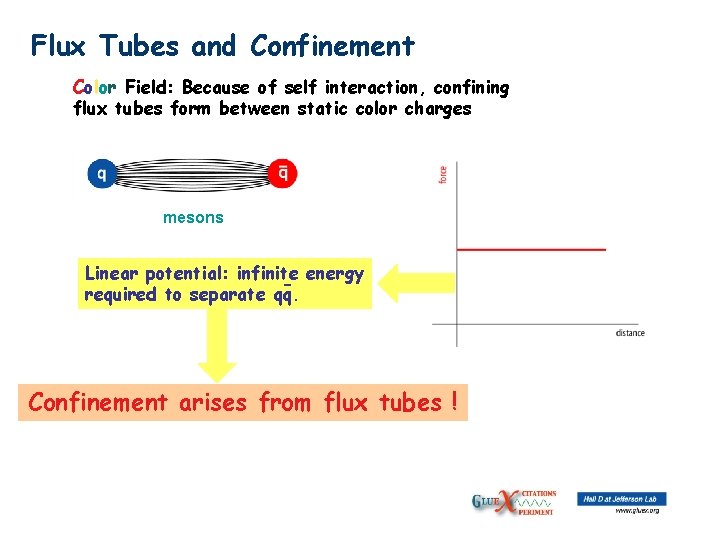
Flux Tubes and Confinement Color Field: Because of self interaction, confining flux tubes form between static color charges mesons Linear potential: infinite energy required to separate qq. Confinement arises from flux tubes !
![Lattice QCD Flux tubes realized From G Bali Vo r Ge V 2 0 Lattice QCD Flux tubes realized From G. Bali Vo( r) [Ge. V] 2. 0](https://slidetodoc.com/presentation_image_h2/7efb86a43acaf481f2d4d998a3fa7f22/image-11.jpg)
Lattice QCD Flux tubes realized From G. Bali Vo( r) [Ge. V] 2. 0 1. 0 linear potential 0. 0 0. 4 0. 8 1. 2 r/fm Lattice “measurement” of quenched static potential 1. 6
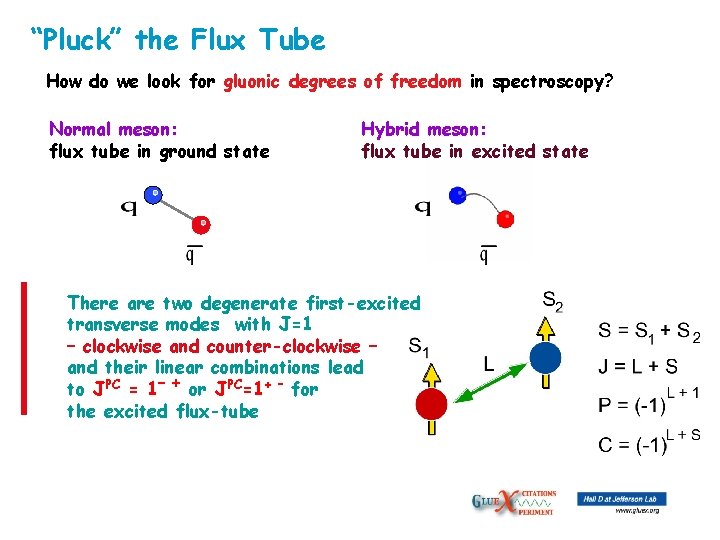
“Pluck” the Flux Tube How do we look for gluonic degrees of freedom in spectroscopy? Normal meson: flux tube in ground state Hybrid meson: flux tube in excited state There are two degenerate first-excited transverse modes with J=1 – clockwise and counter-clockwise – and their linear combinations lead to JPC = 1– + or JPC=1+ – for the excited flux-tube
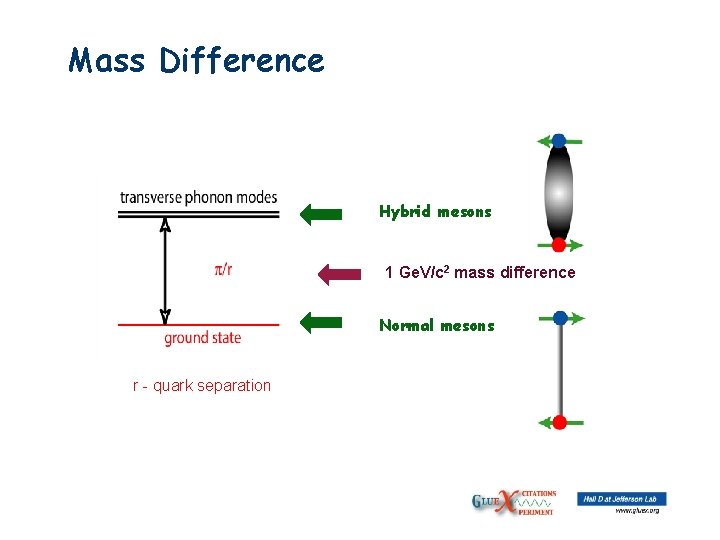
Mass Difference Hybrid mesons 1 Ge. V/c 2 mass difference Normal mesons r - quark separation
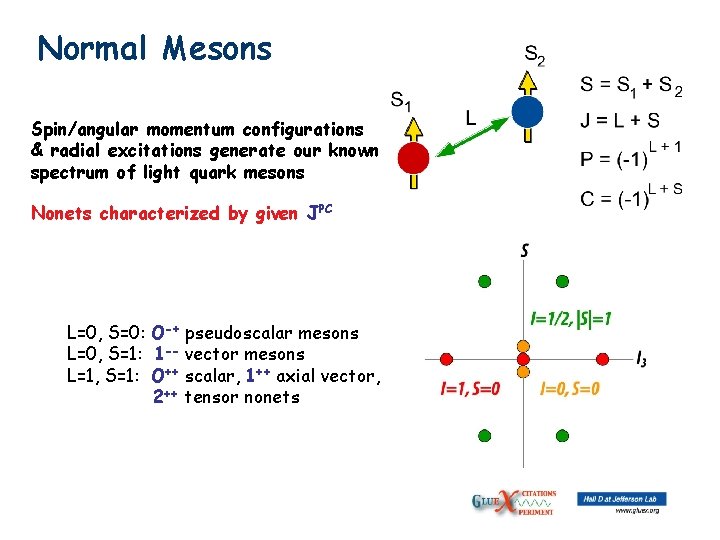
Normal Mesons Spin/angular momentum configurations & radial excitations generate our known spectrum of light quark mesons Nonets characterized by given JPC L=0, S=0: O-+ pseudoscalar mesons L=0, S=1: 1 -- vector mesons L=1, S=1: O++ scalar, 1++ axial vector, 2++ tensor nonets
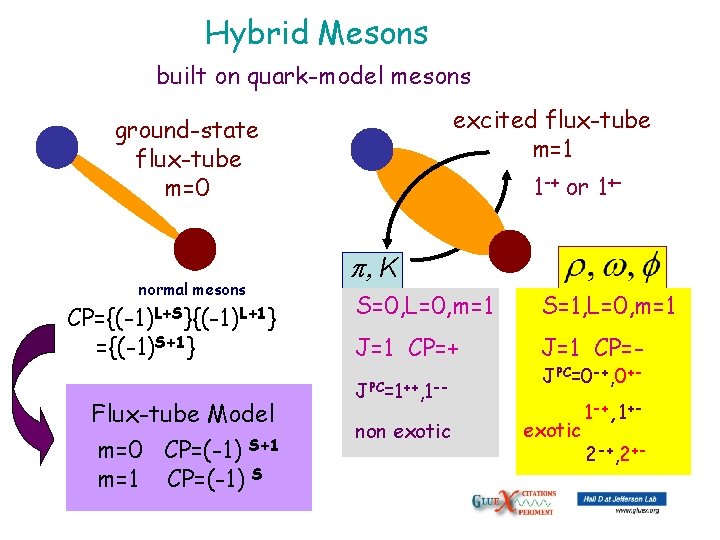
Hybrid Mesons built on quark-model mesons excited flux-tube m=1 ground-state flux-tube m=0 normal mesons CP={(-1)L+S}{(-1)L+1} ={(-1)S+1} Flux-tube Model m=0 CP=(-1) S+1 m=1 CP=(-1) S 1 -+ or 1+- K S=0, L=0, m=1 S=1, L=0, m=1 J=1 CP=+ J=1 CP=- JPC=1++, 1 -non exotic JPC=0 -+, 0+- exotic 1 -+, 1+2 -+, 2+-
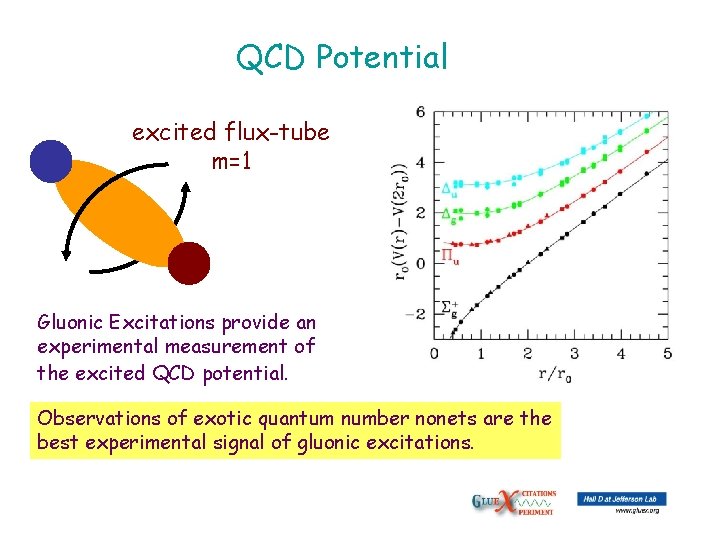
QCD Potential ground-state excited flux-tube m=1 m=0 linear potential Gluonic Excitations provide an experimental measurement of the excited QCD potential. Observations of exotic quantum number nonets are the best experimental signal of gluonic excitations.
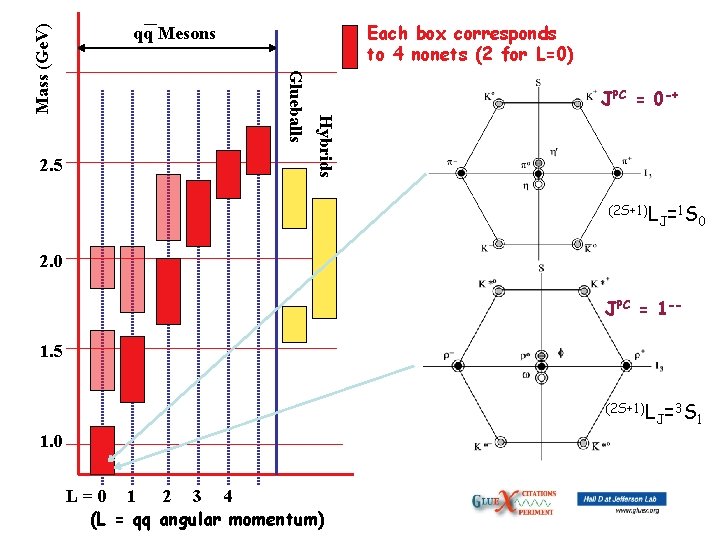
Mass (Ge. V) Map 1 JPC = 0 -+ Hybrids Glueballs 2. 5 Each box corresponds. Meson to 4 nonets (2 for L=0) qq Mesons (2 S+1)L J= 1 S 0 2. 0 JPC = 1 -1. 5 (2 S+1)L 1. 0 L=0 1 2 3 4 (L = qq angular momentum) J= 3 S 1
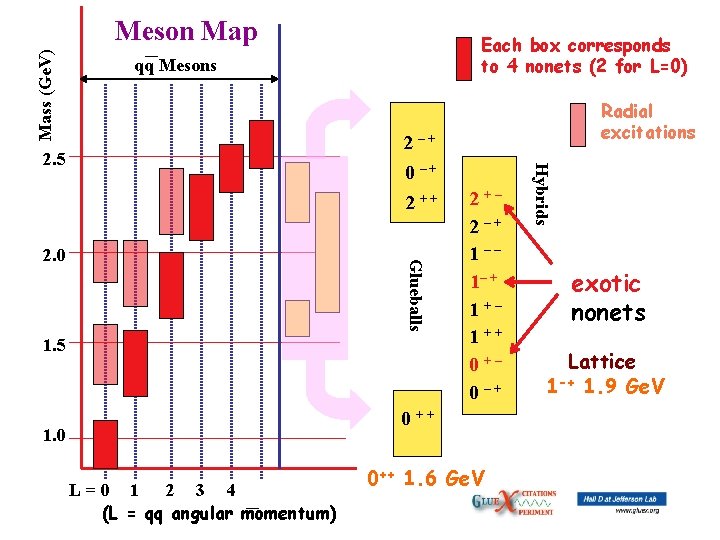
Mass (Ge. V) Meson Map Each box corresponds to 4 nonets (2 for L=0) qq Mesons Radial excitations 2 –+ 0 –+ 2 ++ Glueballs 2. 0 1. 5 2 +– 2 –+ 1 –– 1– + 1 +– 1 ++ 0 +– 0 –+ 0 ++ 1. 0 L=0 1 2 3 4 (L = qq angular momentum) 0++ 1. 6 Ge. V Hybrids 2. 5 exotic nonets Lattice 1 -+ 1. 9 Ge. V
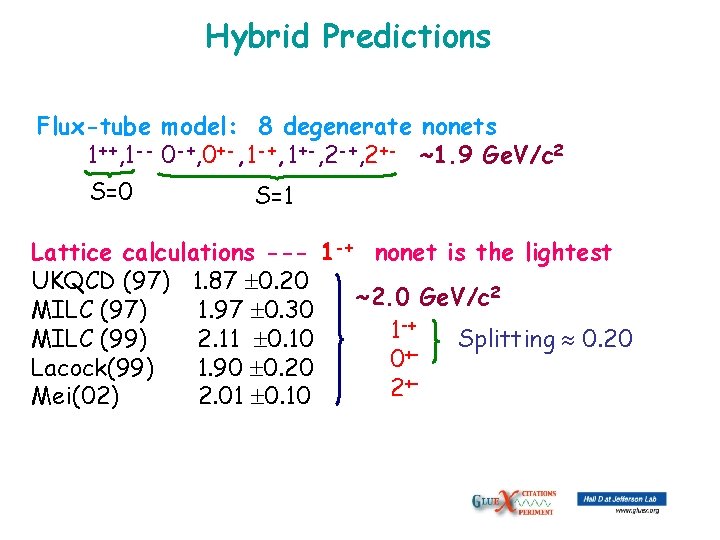
Hybrid Predictions Flux-tube model: 8 degenerate nonets 1++, 1 -- 0 -+, 0+-, 1 -+, 1+-, 2 -+, 2+- ~1. 9 Ge. V/c 2 S=0 S=1 Lattice calculations --- 1 -+ nonet is the lightest UKQCD (97) 1. 87 0. 20 ~2. 0 Ge. V/c 2 MILC (97) 1. 97 0. 30 -+ 1 Splitting 0. 20 MILC (99) 2. 11 0. 10 +0 Lacock(99) 1. 90 0. 20 +2 Mei(02) 2. 01 0. 10
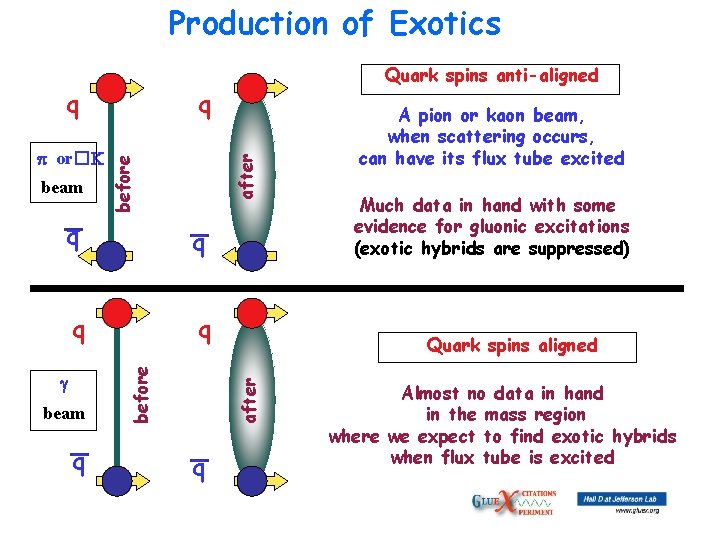
Production of Exotics q _ q beam _ q after q before q _ q A pion or kaon beam, when scattering occurs, can have its flux tube excited Much data in hand with some evidence for gluonic excitations (exotic hybrids are suppressed) Quark spins aligned after beam q before or� Quark spins anti-aligned Almost no data in hand in the mass region where we expect to find exotic hybrids when flux tube is excited
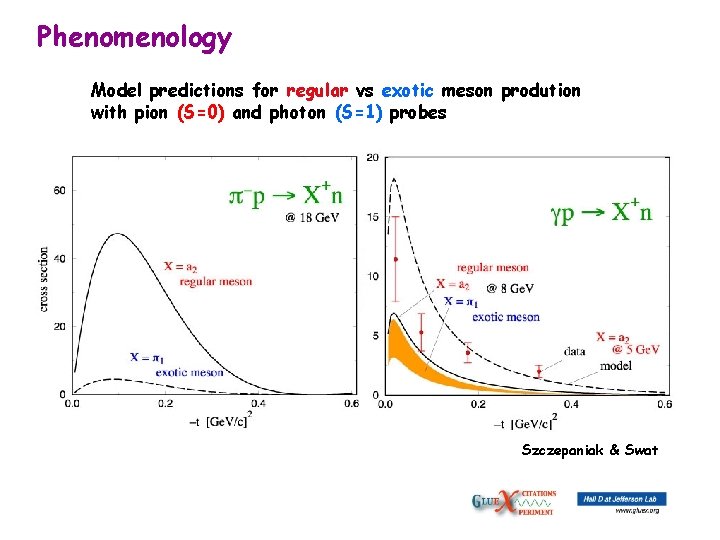
Phenomenology Model predictions for regular vs exotic meson prodution with pion (S=0) and photon (S=1) probes Szczepaniak & Swat
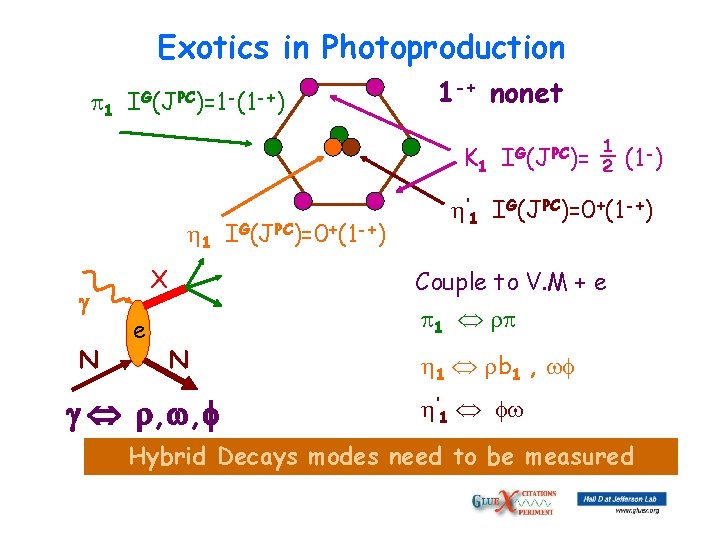
Exotics in Photoproduction 1 IG(JPC)=1 -(1 -+) 1 -+ nonet K 1 IG(JPC)= ½ (1 -) 1 IG(JPC)=0+(1 -+) N X e ’ 1 IG(JPC)=0+(1 -+) Couple to V. M + e 1 r N , , 1 rb 1 , wf ’ 1 fw Hybrid Decays modes need to be measured
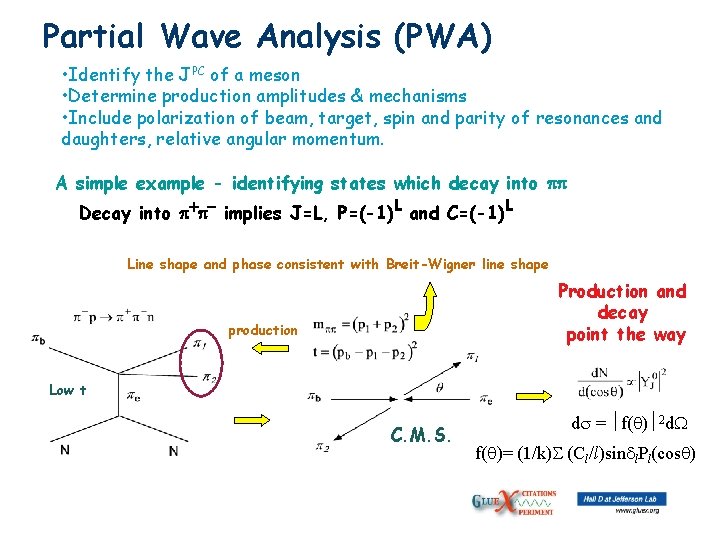
Partial Wave Analysis (PWA) • Identify the JPC of a meson • Determine production amplitudes & mechanisms • Include polarization of beam, target, spin and parity of resonances and daughters, relative angular momentum. A simple example - identifying states which decay into Decay into implies J=L, P=(-1)L and C=(-1)L Line shape and phase consistent with Breit-Wigner line shape Production and decay point the way production Low t C. M. S. d = f( ) 2 d f( )= (1/k) (Cl/l)sin l. Pl(cos )
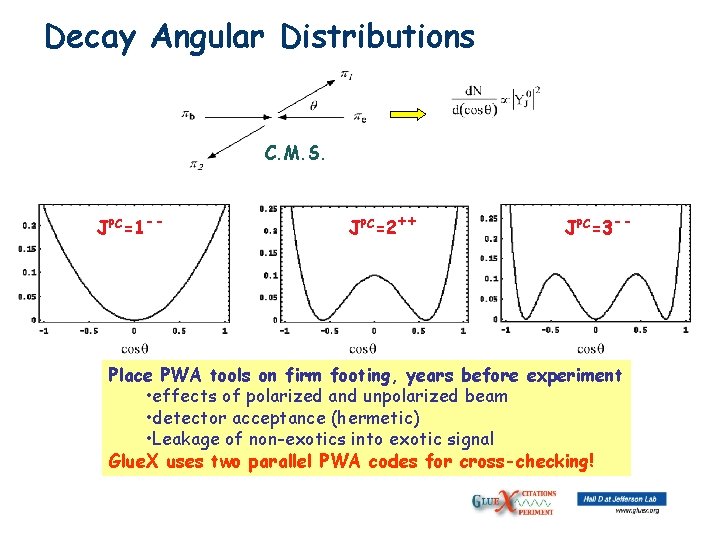
Decay Angular Distributions C. M. S. JPC=1 -- JPC=2++ JPC=3 -- Place PWA tools on firm footing, years before experiment • effects of polarized and unpolarized beam • detector acceptance (hermetic) • Leakage of non-exotics into exotic signal Glue. X uses two parallel PWA codes for cross-checking!
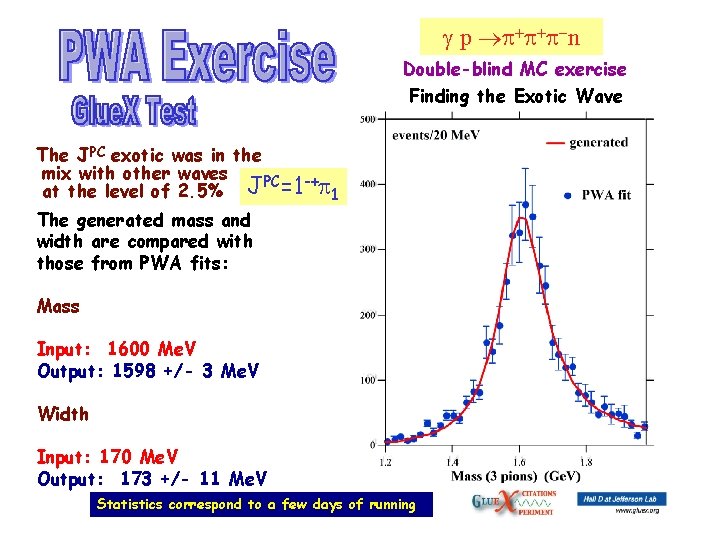
p n Double-blind MC exercise Finding the Exotic Wave The J PC exotic was in the mix with other waves PC -+ at the level of 2. 5% J =1 1 The generated mass and width are compared with those from PWA fits: Mass Input: 1600 Me. V Output: 1598 +/- 3 Me. V Width Input: 170 Me. V Output: 173 +/- 11 Me. V Statistics correspond to a few days of running
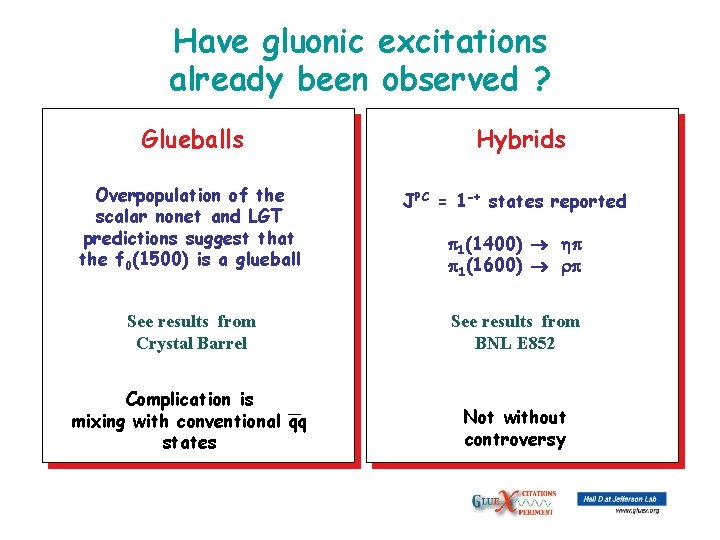
Current Evidence Have gluonic excitations already been observed ? Glueballs Hybrids Overpopulation of the scalar nonet and LGT predictions suggest that the f 0(1500) is a glueball JPC = 1 -+ states reported See results from Crystal Barrel See results from BNL E 852 Complication is mixing with conventional qq states Not without controversy 1(1400) 1(1600)
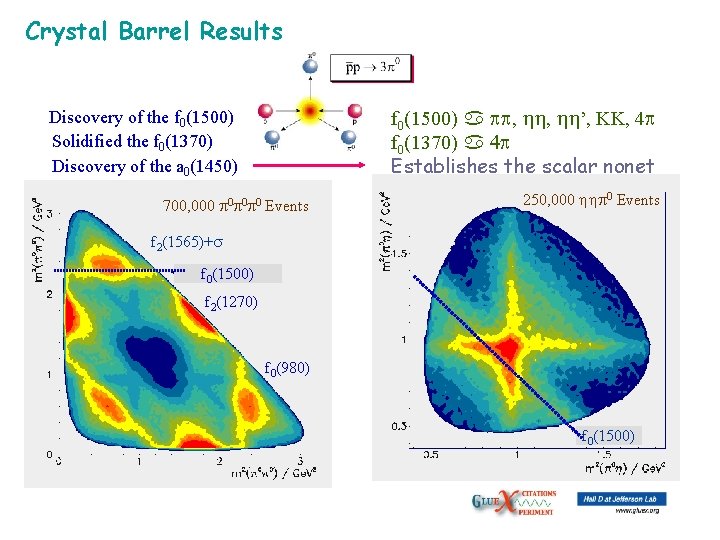
Crystal Barrel Results f 0(1500) a , , ’, KK, 4 f 0(1370) a 4 Establishes the scalar nonet Discovery of the f 0(1500) Solidified the f 0(1370) Discovery of the a 0(1450) 700, 000 0 Events 250, 000 0 Events f 2(1565)+ f 0(1500) f 2(1270) f 0(980) f 0(1500)
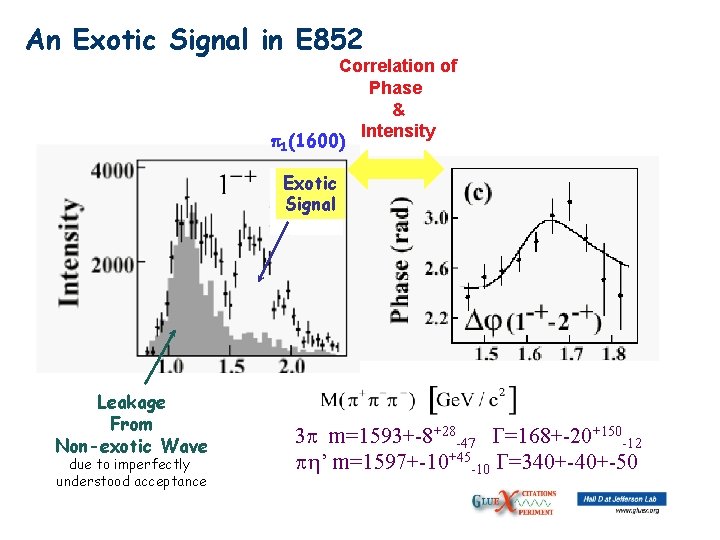
An Exotic Signal in E 852 Correlation of Phase & Intensity 1(1600) Exotic Signal Leakage From Non-exotic Wave due to imperfectly understood acceptance 3 m=1593+-8+28 -47 G=168+-20+150 -12 ’ m=1597+-10+45 -10 G=340+-50
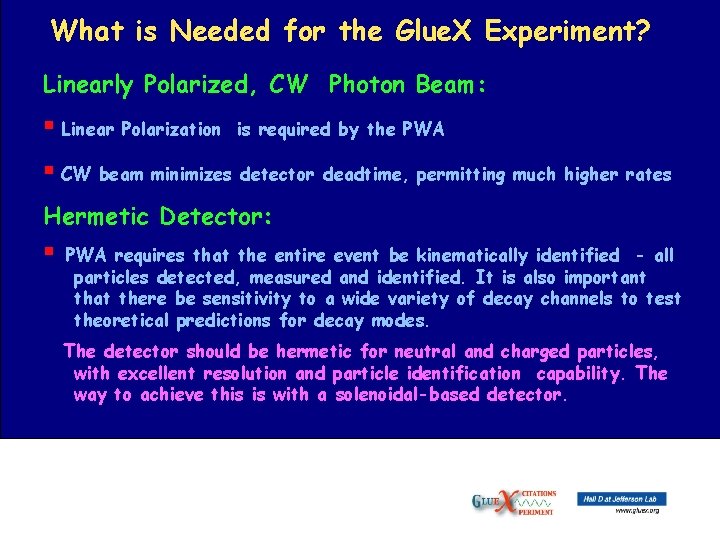
What is Needed for the Glue. X Experiment? Linearly Polarized, CW Photon Beam: § Linear Polarization is required by the PWA § CW beam minimizes detector deadtime, permitting much higher rates Hermetic Detector: § PWA requires that the entire event be kinematically identified - all particles detected, measured and identified. It is also important that there be sensitivity to a wide variety of decay channels to test theoretical predictions for decay modes. The detector should be hermetic for neutral and charged particles, with excellent resolution and particle identification capability. The way to achieve this is with a solenoidal-based detector.
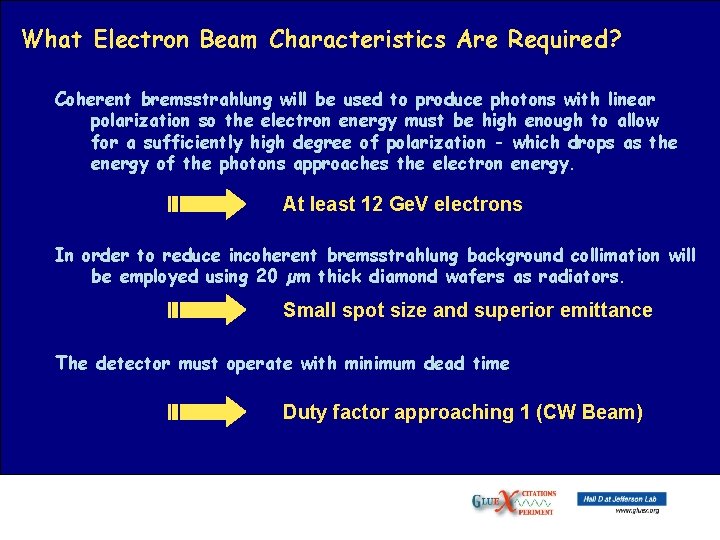
What Electron Beam Characteristics Are Required? Coherent bremsstrahlung will be used to produce photons with linear polarization so the electron energy must be high enough to allow for a sufficiently high degree of polarization - which drops as the energy of the photons approaches the electron energy. At least 12 Ge. V electrons In order to reduce incoherent bremsstrahlung background collimation will be employed using 20 µm thick diamond wafers as radiators. Small spot size and superior emittance The detector must operate with minimum dead time Duty factor approaching 1 (CW Beam)
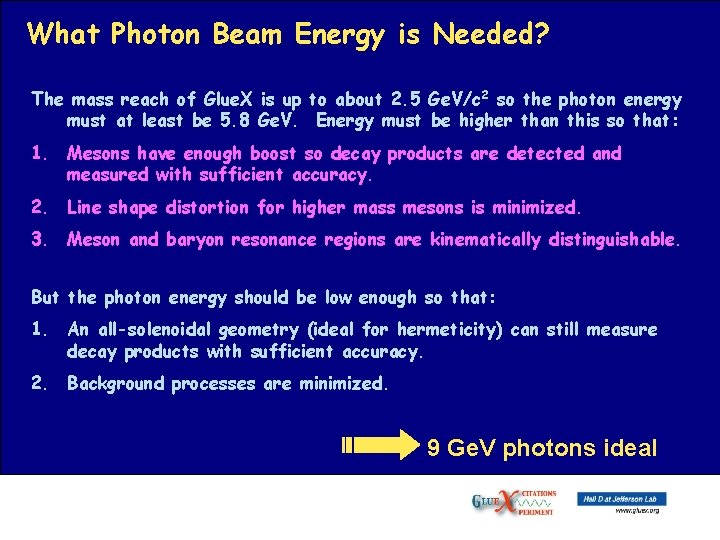
What Photon Beam Energy is Needed? The mass reach of Glue. X is up to about 2. 5 Ge. V/c 2 so the photon energy must at least be 5. 8 Ge. V. Energy must be higher than this so that: 1. Mesons have enough boost so decay products are detected and measured with sufficient accuracy. 2. Line shape distortion for higher mass mesons is minimized. 3. Meson and baryon resonance regions are kinematically distinguishable. But the photon energy should be low enough so that: 1. An all-solenoidal geometry (ideal for hermeticity) can still measure decay products with sufficient accuracy. 2. Background processes are minimized. 9 Ge. V photons ideal
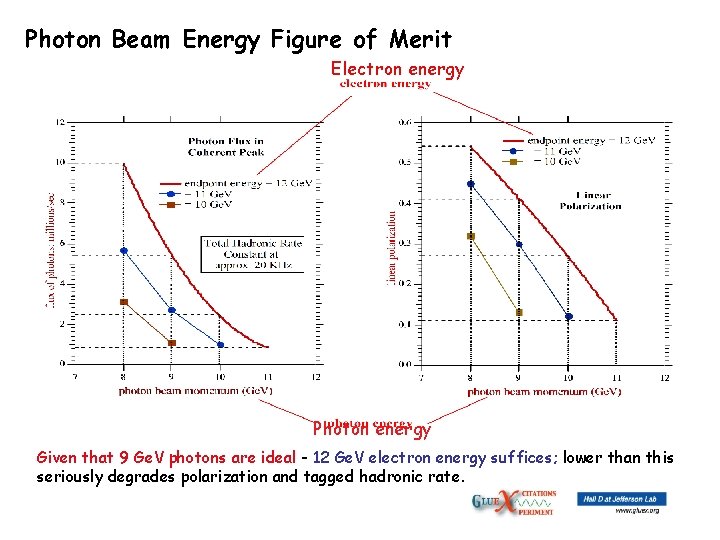
Photon Beam Energy Figure of Merit Electron energy Photon energy Given that 9 Ge. V photons are ideal - 12 Ge. V electron energy suffices; lower than this seriously degrades polarization and tagged hadronic rate.
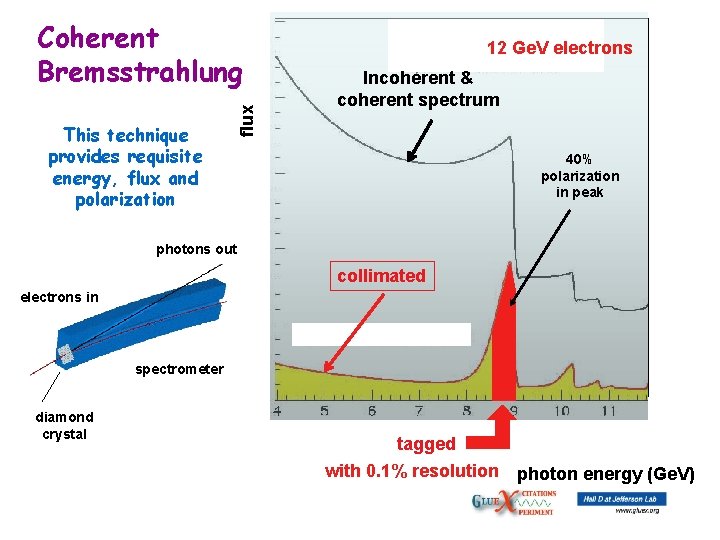
This technique provides requisite energy, flux and polarization flux Coherent Bremsstrahlung 12 Ge. V electrons Incoherent & coherent spectrum 40% polarization in peak photons out collimated electrons in spectrometer diamond crystal tagged with 0. 1% resolution photon energy (Ge. V)
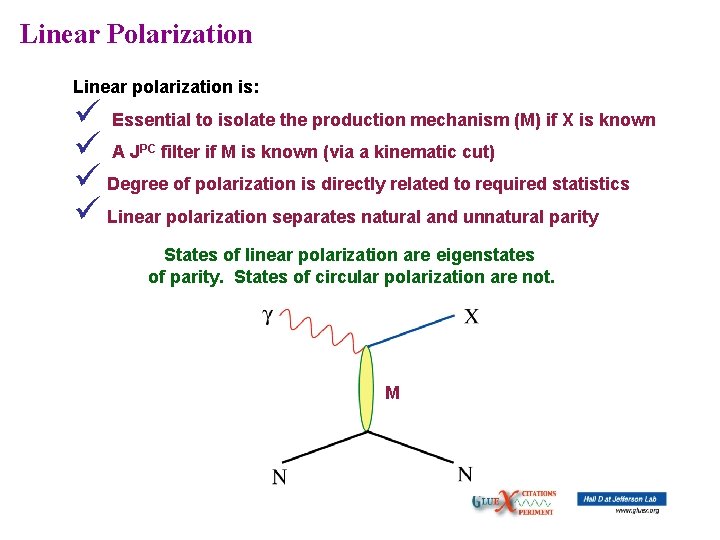
Linear Polarization Linear polarization is: ü Essential to isolate the production mechanism (M) if X is known ü A J filter if M is known (via a kinematic cut) ü Degree of polarization is directly related to required statistics ü Linear polarization separates natural and unnatural parity PC States of linear polarization are eigenstates of parity. States of circular polarization are not. M
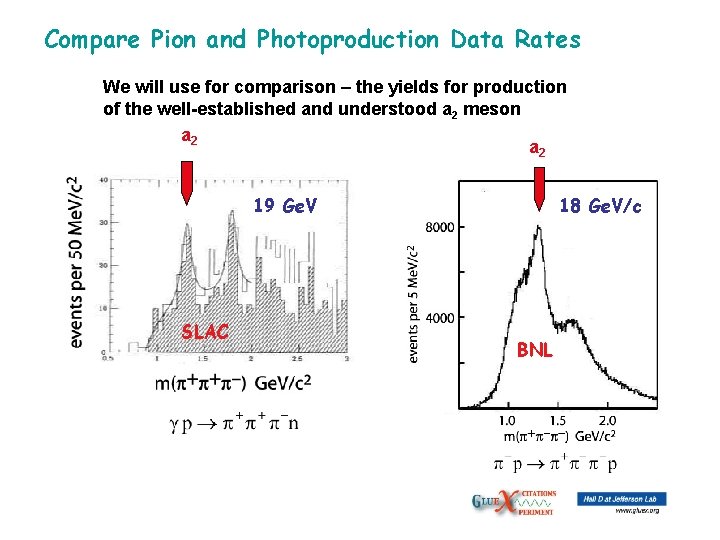
Compare Pion and Photoproduction Data Rates We will use for comparison – the yields for production of the well-established and understood a 2 meson a 2 19 Ge. V SLAC 18 Ge. V/c BNL
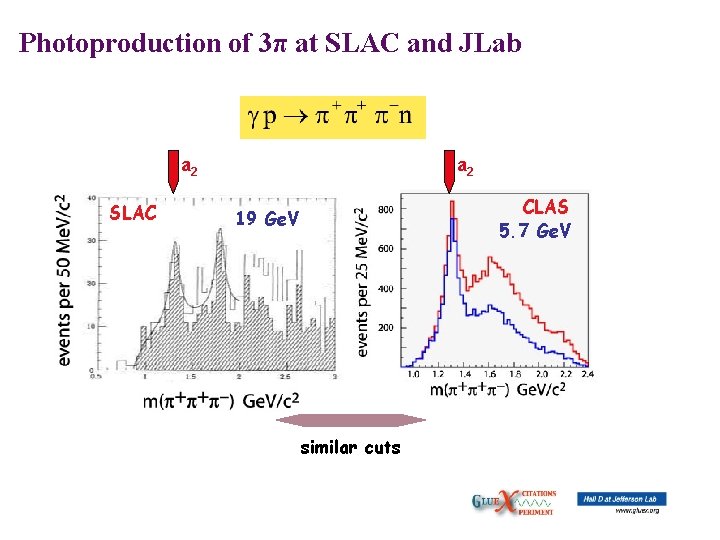
Photoproduction of 3π at SLAC and JLab a 2 SLAC a 2 CLAS 5. 7 Ge. V 19 Ge. V similar cuts
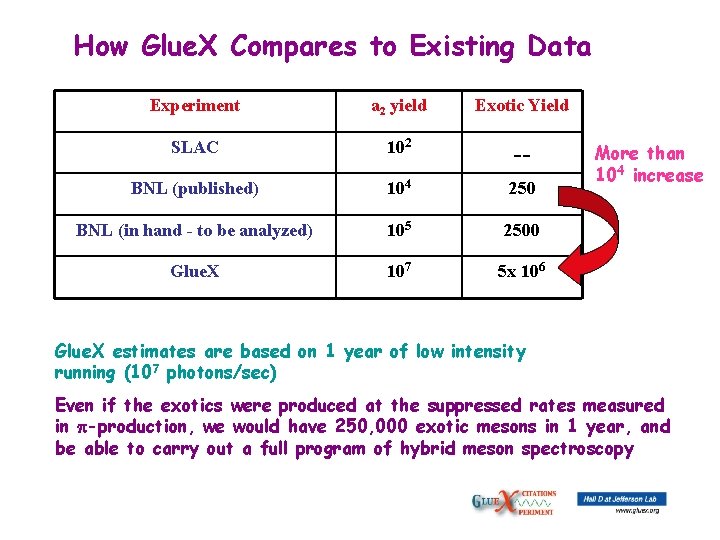
How Glue. X Compares to Existing Data Experiment a 2 yield Exotic Yield SLAC 102 -- BNL (published) 104 250 BNL (in hand - to be analyzed) 105 2500 Glue. X 107 5 x 106 More than 104 increase Glue. X estimates are based on 1 year of low intensity running (107 photons/sec) Even if the exotics were produced at the suppressed rates measured in -production, we would have 250, 000 exotic mesons in 1 year, and be able to carry out a full program of hybrid meson spectroscopy
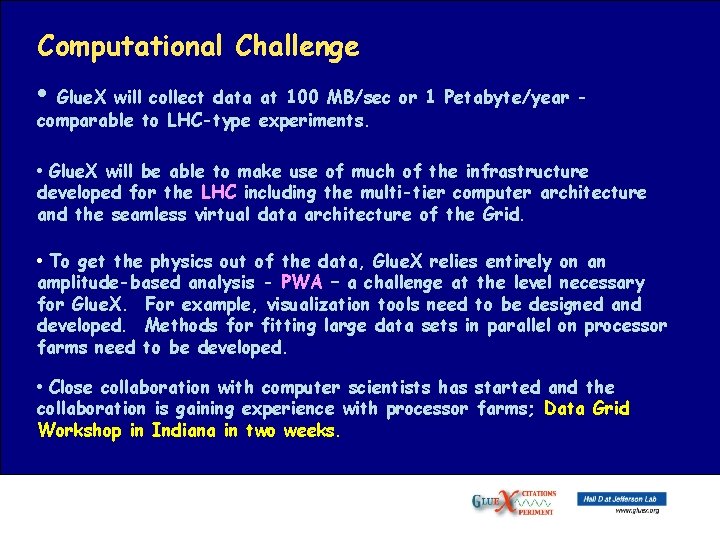
Computational Challenge • Glue. X will collect data at 100 MB/sec or 1 Petabyte/year comparable to LHC-type experiments. • Glue. X will be able to make use of much of the infrastructure developed for the LHC including the multi-tier computer architecture and the seamless virtual data architecture of the Grid. • To get the physics out of the data, Glue. X relies entirely on an amplitude-based analysis - PWA – a challenge at the level necessary for Glue. X. For example, visualization tools need to be designed and developed. Methods for fitting large data sets in parallel on processor farms need to be developed. • Close collaboration with computer scientists has started and the collaboration is gaining experience with processor farms; Data Grid Workshop in Indiana in two weeks.
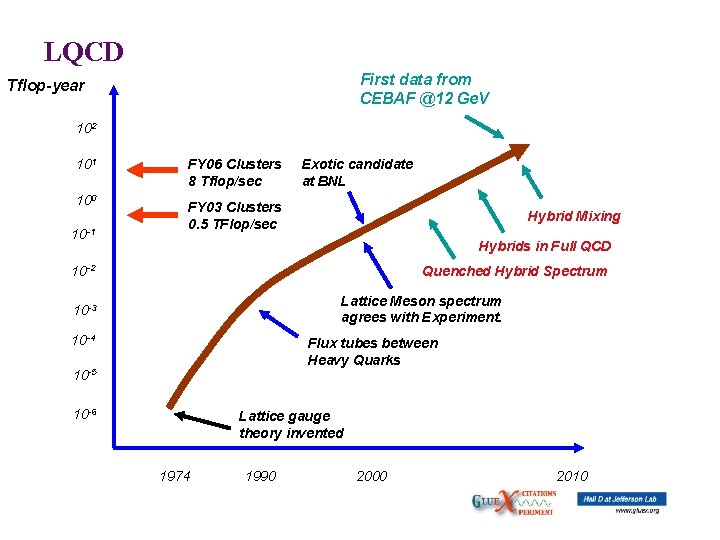
LQCD First data from CEBAF @12 Ge. V Tflop-year 102 101 100 10 -1 FY 06 Clusters 8 Tflop/sec Exotic candidate at BNL FY 03 Clusters 0. 5 TFlop/sec Hybrid Mixing Hybrids in Full QCD 10 -2 Quenched Hybrid Spectrum Lattice Meson spectrum agrees with Experiment. 10 -3 10 -4 Flux tubes between Heavy Quarks 10 -5 10 -6 Lattice gauge theory invented 1974 1990 2000 2010
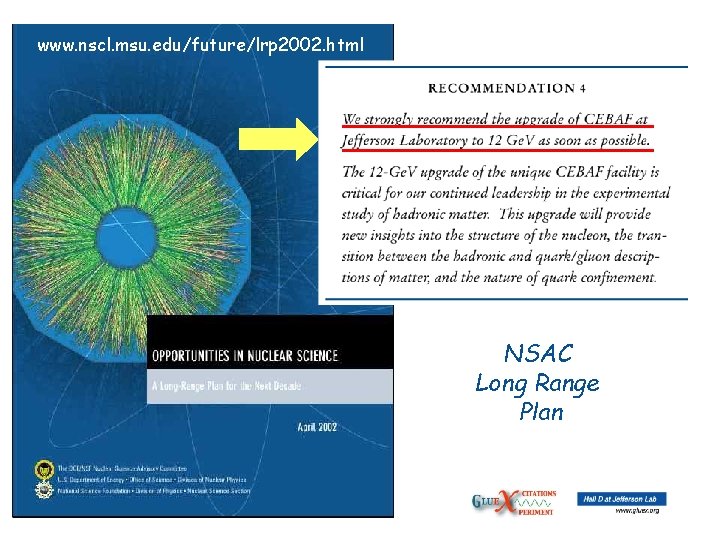
www. nscl. msu. edu/future/lrp 2002. html LRP NSAC Long Range Plan
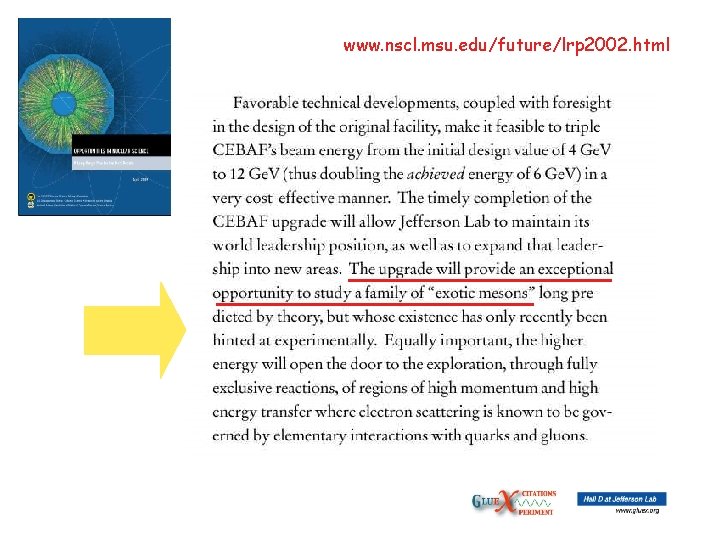
www. nscl. msu. edu/future/lrp 2002. html LRP
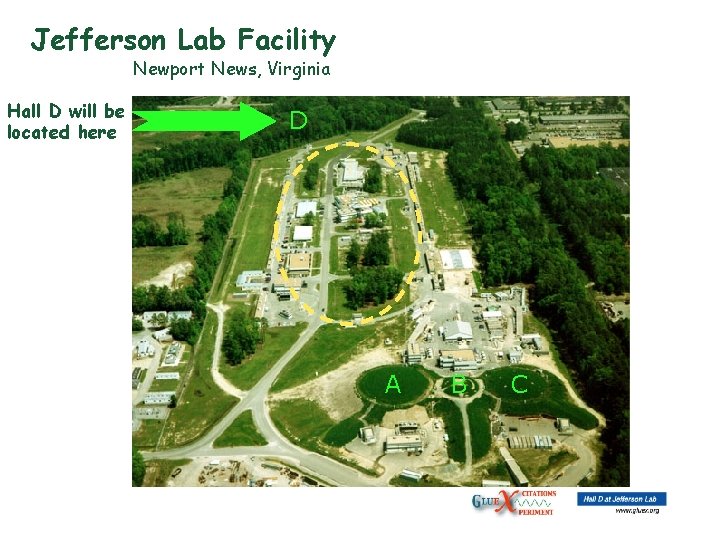
Jefferson Lab Facility Newport News, Virginia Hall D will be located here D A B C
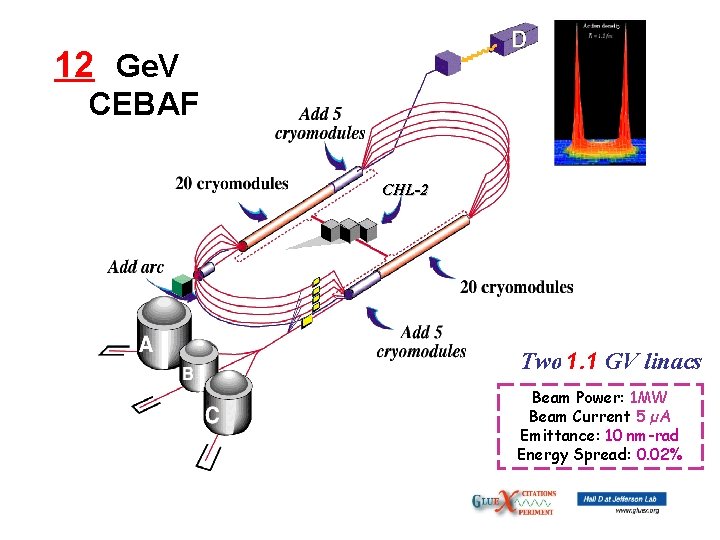
116 Ge. V 12 Upgrade magnets and power supplies CEBAF CHL-2 Two 1. 1 0. 6 GV linacs Beam Power: 1 MW Beam Current 5 µA Emittance: 10 nm-rad Energy Spread: 0. 02%
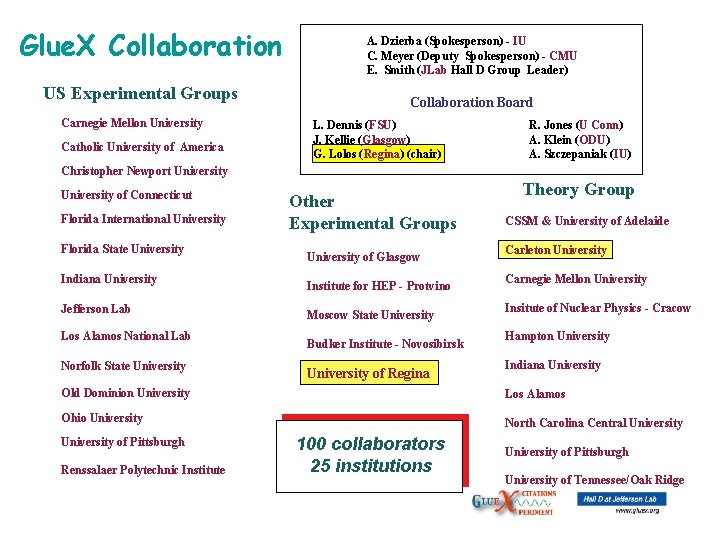
Glue. X Collaboration US Experimental Groups Carnegie Mellon University Catholic University of America A. Dzierba (Spokesperson) - IU C. Meyer (Deputy Spokesperson) - CMU E. Smith (JLab Hall D Group Leader) Collaboration Board L. Dennis (FSU) J. Kellie (Glasgow) G. Lolos (Regina) (chair) R. Jones (U Conn) A. Klein (ODU) A. Szczepaniak (IU) Christopher Newport University of Connecticut Florida International University Florida State University Indiana University Jefferson Lab Los Alamos National Lab Norfolk State University Other Experimental Groups University of Glasgow Institute for HEP - Protvino Moscow State University Budker Institute - Novosibirsk University of Regina Theory Group CSSM & University of Adelaide Carleton University Carnegie Mellon University Insitute of Nuclear Physics - Cracow Hampton University Indiana University Old Dominion University Los Alamos Ohio University North Carolina Central University of Pittsburgh Renssalaer Polytechnic Institute 100 collaborators 25 institutions University of Pittsburgh University of Tennessee/Oak Ridge
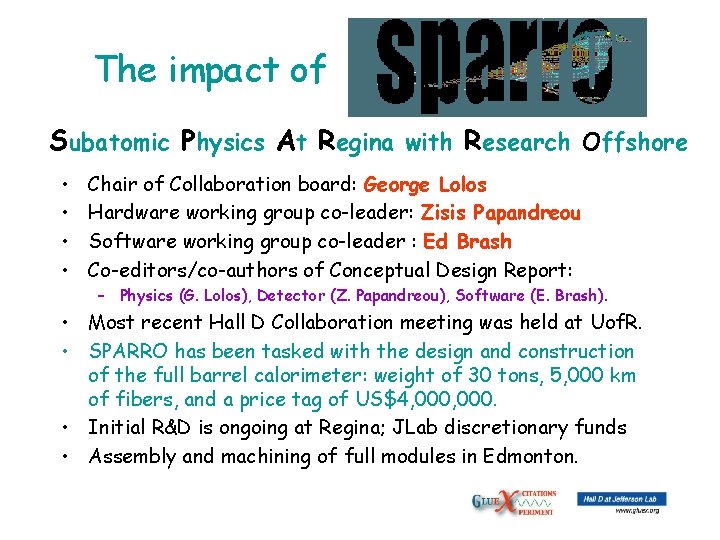
The impact of Subatomic Physics At Regina with Research Offshore • • Chair of Collaboration board: George Lolos Hardware working group co-leader: Zisis Papandreou Software working group co-leader : Ed Brash Co-editors/co-authors of Conceptual Design Report: – Physics (G. Lolos), Detector (Z. Papandreou), Software (E. Brash). • Most recent Hall D Collaboration meeting was held at Uof. R. • SPARRO has been tasked with the design and construction of the full barrel calorimeter: weight of 30 tons, 5, 000 km of fibers, and a price tag of US$4, 000. • Initial R&D is ongoing at Regina; JLab discretionary funds • Assembly and machining of full modules in Edmonton.
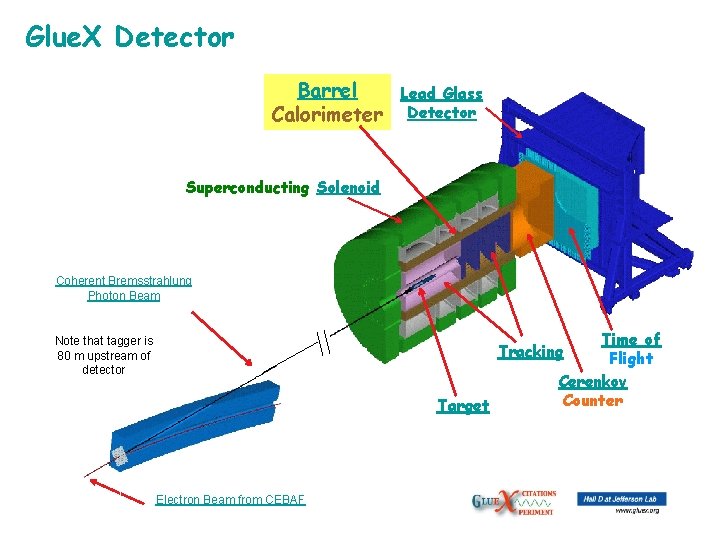
Glue. X Detector Barrel Calorimeter Lead Glass Detector Superconducting Solenoid Coherent Bremsstrahlung Photon Beam Note that tagger is 80 m upstream of detector Time of Flight Cerenkov Counter Tracking Target Electron Beam from CEBAF
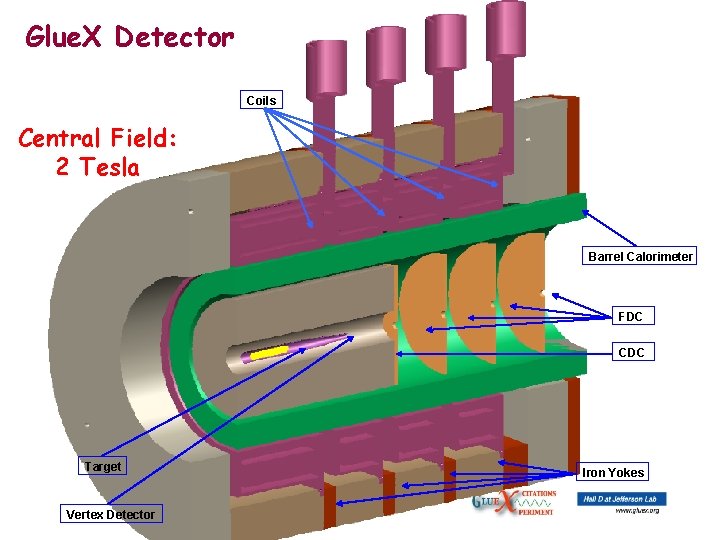
Glue. X Detector Coils Central Field: 2 Tesla Barrel Calorimeter FDC CDC Target Vertex Detector Iron Yokes
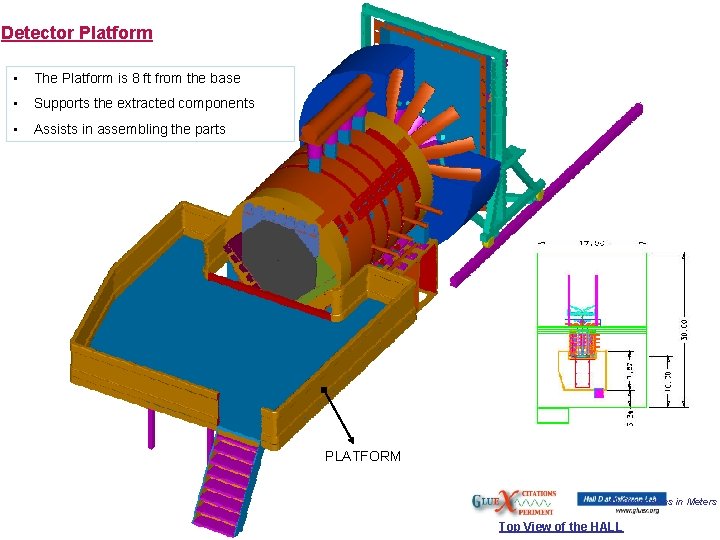
Detector Platform • The Platform is 8 ft from the base • Supports the extracted components • Assists in assembling the parts PLATFORM All Dimensions in Meters Top View of the HALL
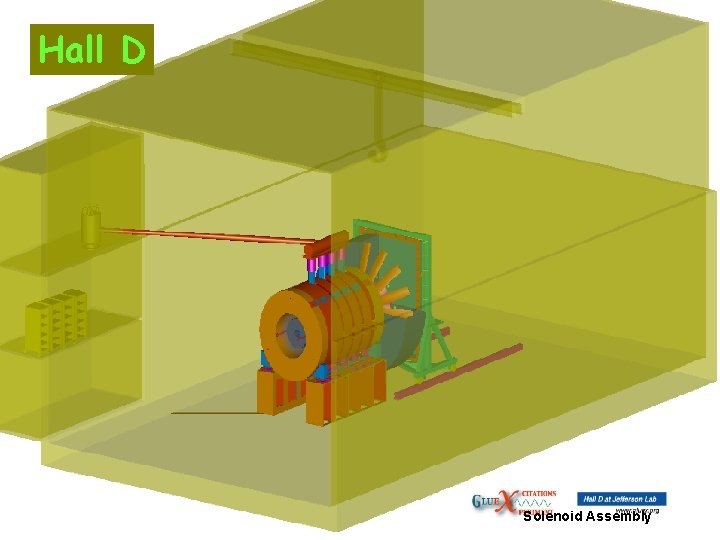
Hall D Solenoid Assembly
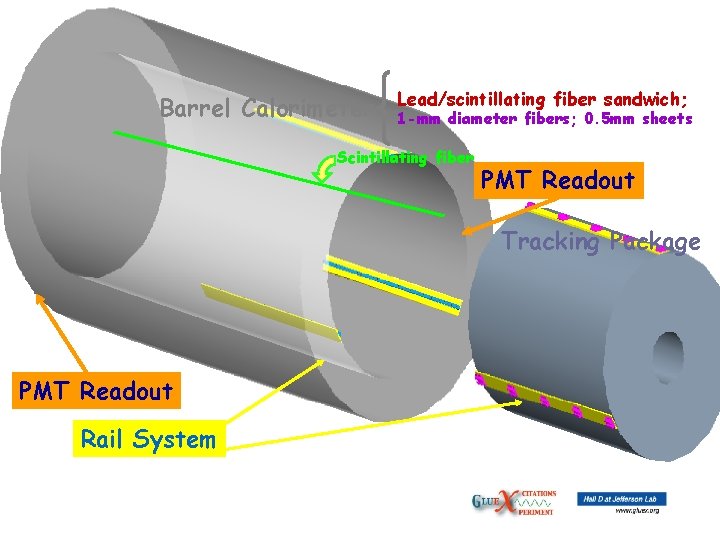
Barrel Calorimeter Lead/scintillating fiber sandwich; 1 -mm diameter fibers; 0. 5 mm sheets Scintillating fiber PMT Readout Tracking Package PMT Readout Rail System
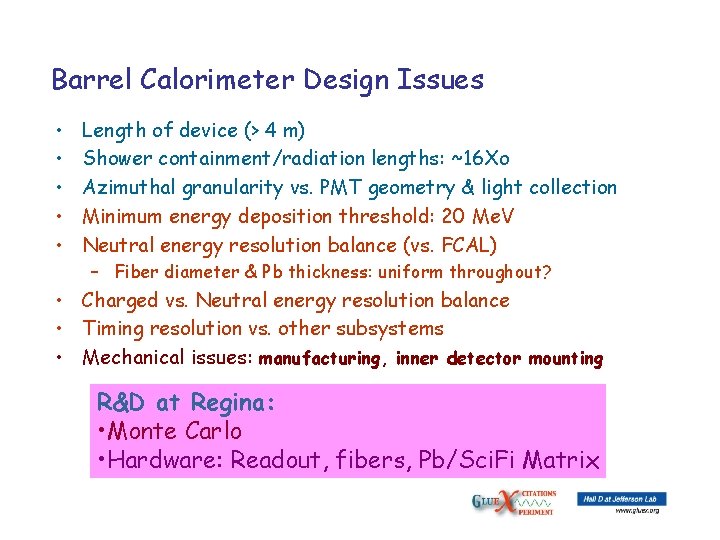
Barrel Calorimeter Design Issues • • • Length of device (> 4 m) Shower containment/radiation lengths: ~16 Xo Azimuthal granularity vs. PMT geometry & light collection Minimum energy deposition threshold: 20 Me. V Neutral energy resolution balance (vs. FCAL) – Fiber diameter & Pb thickness: uniform throughout? • Charged vs. Neutral energy resolution balance • Timing resolution vs. other subsystems • Mechanical issues: manufacturing, inner detector mounting R&D at Regina: • Monte Carlo • Hardware: Readout, fibers, Pb/Sci. Fi Matrix
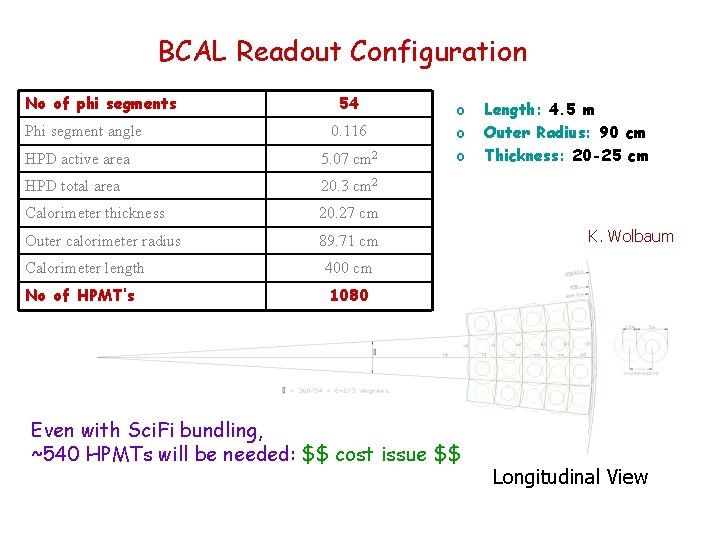
BCAL Readout Configuration No of phi segments Phi segment angle 54 0. 116 HPD active area 5. 07 cm 2 HPD total area 20. 3 cm 2 Calorimeter thickness 20. 27 cm Outer calorimeter radius 89. 71 cm Calorimeter length 400 cm No of HPMT’s 1080 o o o Even with Sci. Fi bundling, ~540 HPMTs will be needed: $$ cost issue $$ Length: 4. 5 m Outer Radius: 90 cm Thickness: 20 -25 cm K. Wolbaum Longitudinal View
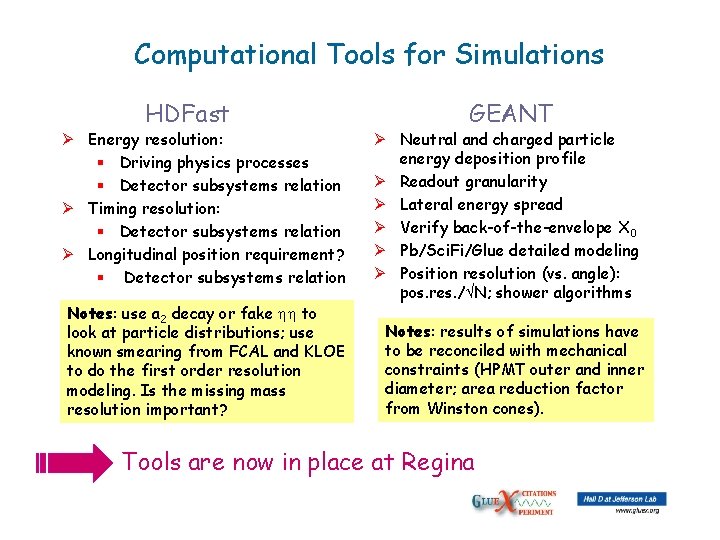
Computational Tools for Simulations HDFast Ø Energy resolution: § Driving physics processes § Detector subsystems relation Ø Timing resolution: § Detector subsystems relation Ø Longitudinal position requirement? § Detector subsystems relation Notes: use a 2 decay or fake to look at particle distributions; use known smearing from FCAL and KLOE to do the first order resolution modeling. Is the missing mass resolution important? GEANT Ø Neutral and charged particle energy deposition profile Ø Readout granularity Ø Lateral energy spread Ø Verify back-of-the-envelope X 0 Ø Pb/Sci. Fi/Glue detailed modeling Ø Position resolution (vs. angle): pos. res. / N; shower algorithms Notes: results of simulations have to be reconciled with mechanical constraints (HPMT outer and inner diameter; area reduction factor from Winston cones). Tools are now in place at Regina
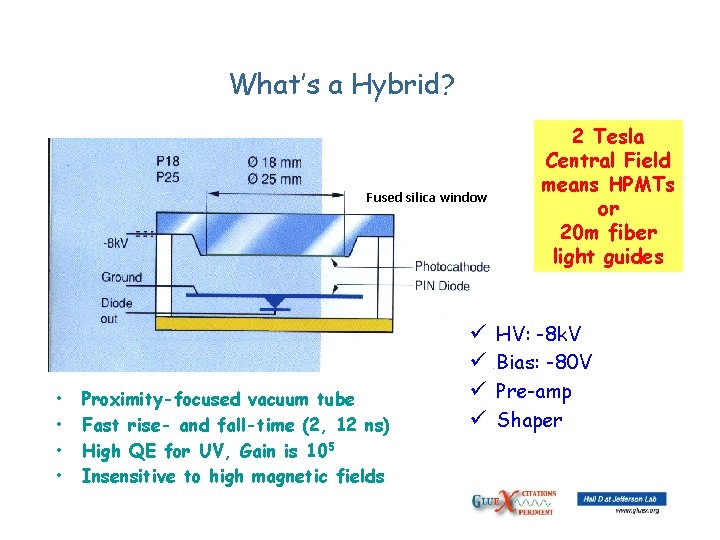
What’s a Hybrid? Fused silica window • • Proximity-focused vacuum tube Fast rise- and fall-time (2, 12 ns) High QE for UV, Gain is 105 Insensitive to high magnetic fields ü ü 2 Tesla Central Field means HPMTs or 20 m fiber light guides HV: -8 k. V Bias: -80 V Pre-amp Shaper
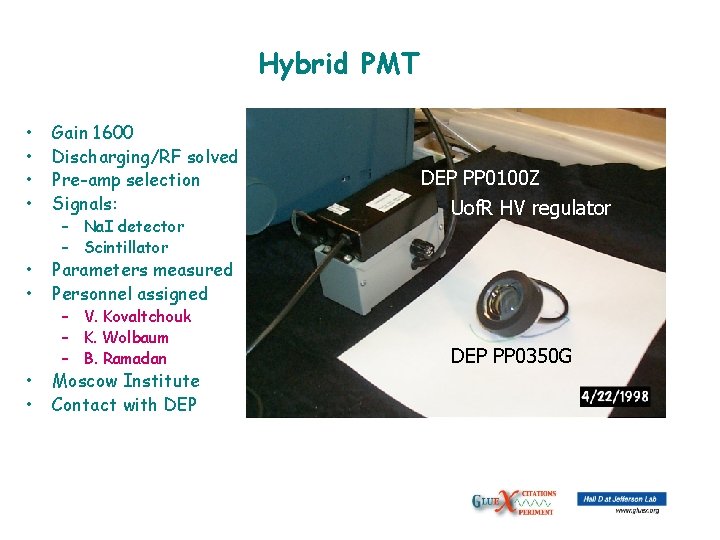
Hybrid PMT • • Gain 1600 Discharging/RF solved Pre-amp selection Signals: • • Parameters measured Personnel assigned • • – Na. I detector – Scintillator – V. Kovaltchouk – K. Wolbaum – B. Ramadan Moscow Institute Contact with DEP PP 0100 Z Uof. R HV regulator DEP PP 0350 G
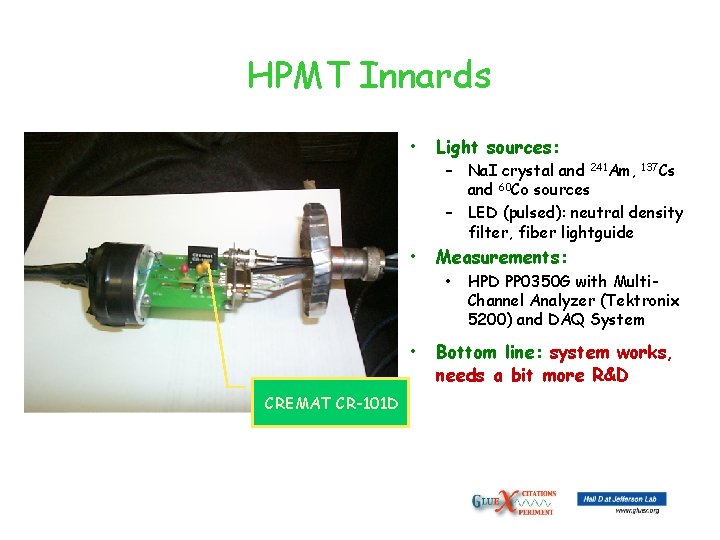
HPMT Innards CREMAT CR-101 D • Light sources: • Measurements: • Bottom line: system works, needs a bit more R&D – Na. I crystal and 241 Am, 137 Cs and 60 Co sources – LED (pulsed): neutral density filter, fiber lightguide • HPD PP 0350 G with Multi. Channel Analyzer (Tektronix 5200) and DAQ System
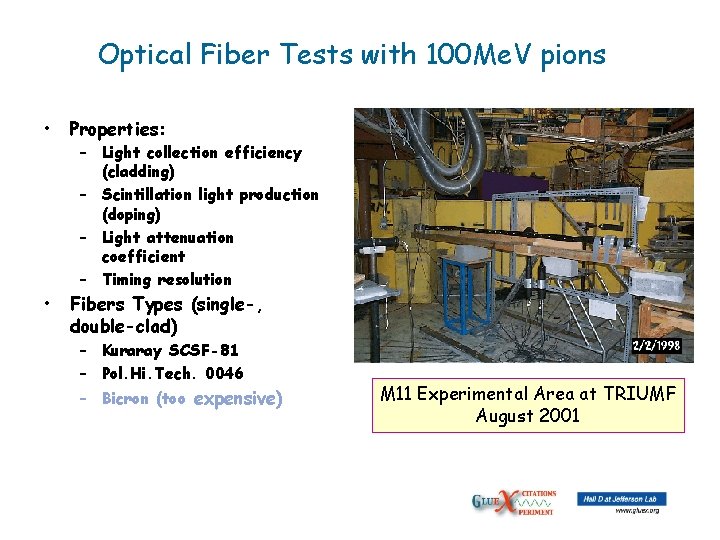
Optical Fiber Tests with 100 Me. V pions • Properties: • Fibers Types (single-, double-clad) – Light collection efficiency (cladding) – Scintillation light production (doping) – Light attenuation coefficient – Timing resolution – Kuraray SCSF-81 – Pol. Hi. Tech. 0046 – Bicron (too expensive) M 11 Experimental Area at TRIUMF August 2001
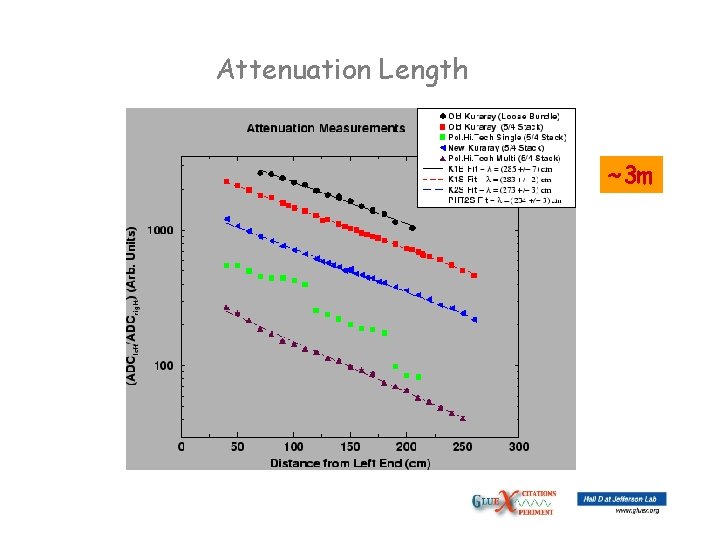
Attenuation Length ~3 m
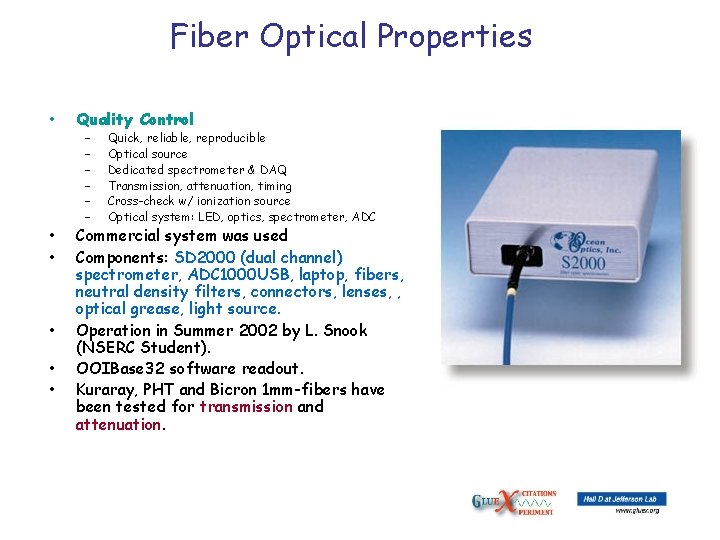
Fiber Optical Properties • Quality Control • • Commercial system was used Components: SD 2000 (dual channel) spectrometer, ADC 1000 USB, laptop, fibers, neutral density filters, connectors, lenses, , optical grease, light source. Operation in Summer 2002 by L. Snook (NSERC Student). OOIBase 32 software readout. Kuraray, PHT and Bicron 1 mm-fibers have been tested for transmission and attenuation. • • • – – – Quick, reliable, reproducible Optical source Dedicated spectrometer & DAQ Transmission, attenuation, timing Cross-check w/ ionization source Optical system: LED, optics, spectrometer, ADC
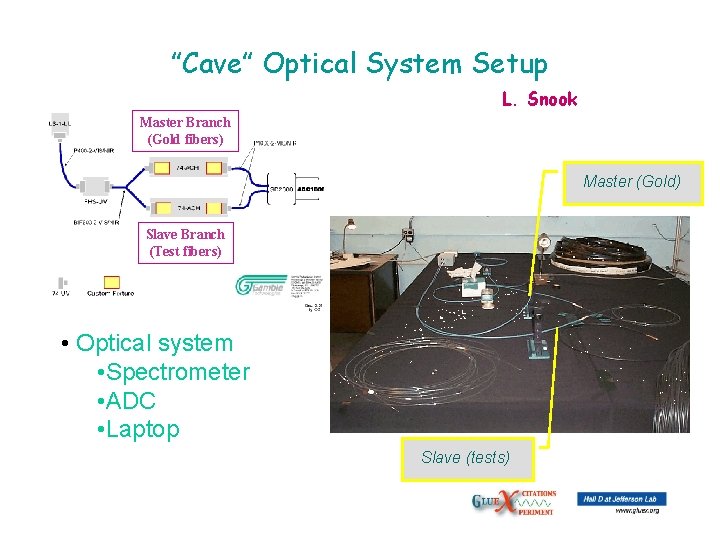
”Cave” Optical System Setup L. Snook Master Branch (Gold fibers) Master (Gold) Slave Branch (Test fibers) • Optical system • Spectrometer • ADC • Laptop Slave (tests)
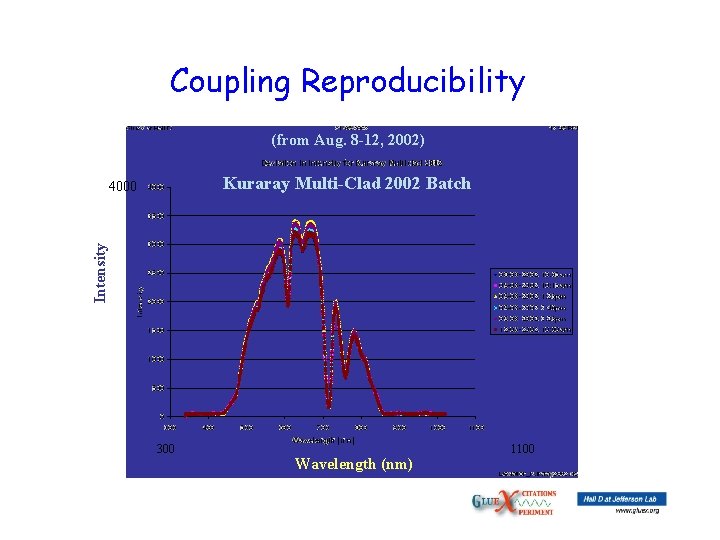
Coupling Reproducibility (from Aug. 8 -12, 2002) Kuraray Multi-Clad 2002 Batch Intensity 4000 300 Wavelength (nm) 1100
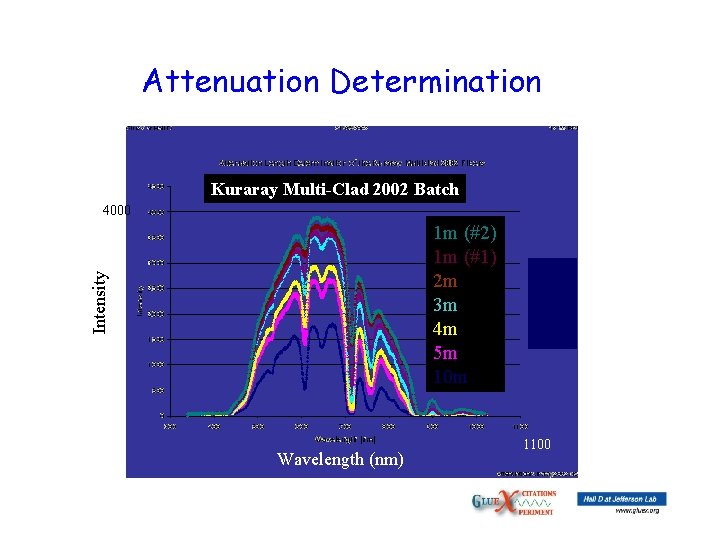
Attenuation Determination Kuraray Multi-Clad 2002 Batch 4000 Intensity 1 m (#2) 1 m (#1) 2 m 3 m 4 m 5 m 10 m Wavelength (nm) 1100
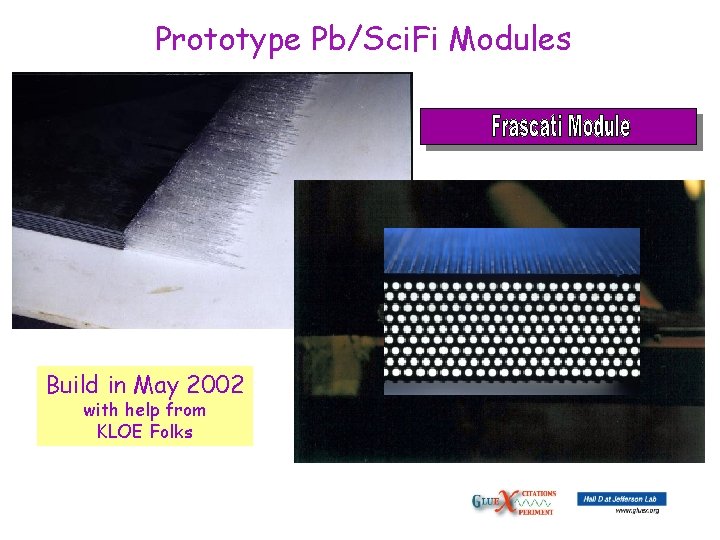
Prototype Pb/Sci. Fi Modules Build in May 2002 with help from KLOE Folks
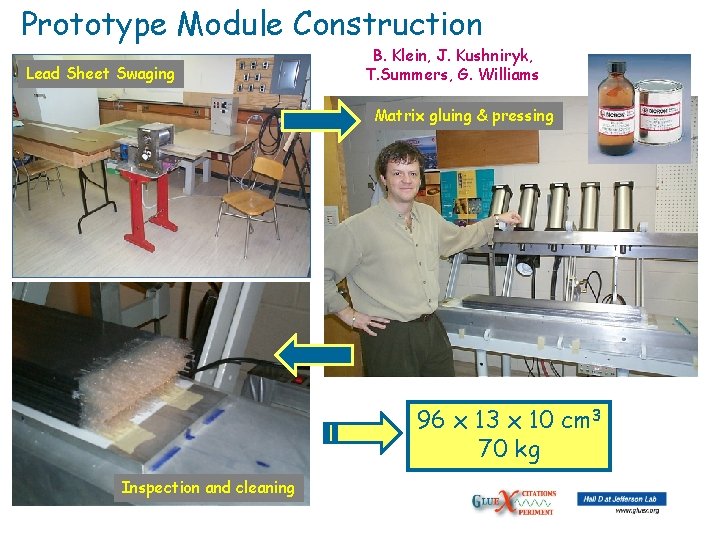
Prototype Module Construction Lead Sheet Swaging B. Klein, J. Kushniryk, T. Summers, G. Williams Matrix gluing & pressing 96 x 13 x 10 cm 3 70 kg Inspection and cleaning
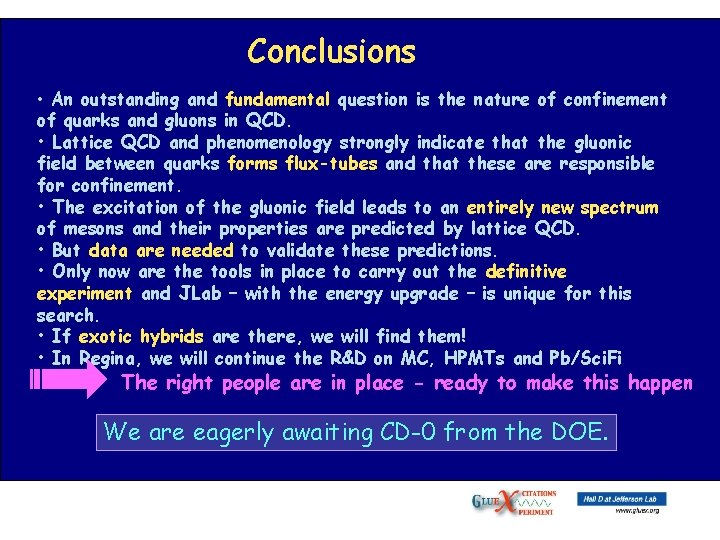
Conclusions • An outstanding and fundamental question is the nature of confinement of quarks and gluons in QCD. • Lattice QCD and phenomenology strongly indicate that the gluonic field between quarks forms flux-tubes and that these are responsible for confinement. • The excitation of the gluonic field leads to an entirely new spectrum of mesons and their properties are predicted by lattice QCD. • But data are needed to validate these predictions. • Only now are the tools in place to carry out the definitive experiment and JLab – with the energy upgrade – is unique for this search. • If exotic hybrids are there, we will find them! • In Regina, we will continue the R&D on MC, HPMTs and Pb/Sci. Fi The right people are in place - ready to make this happen We are eagerly awaiting CD-0 from the DOE.
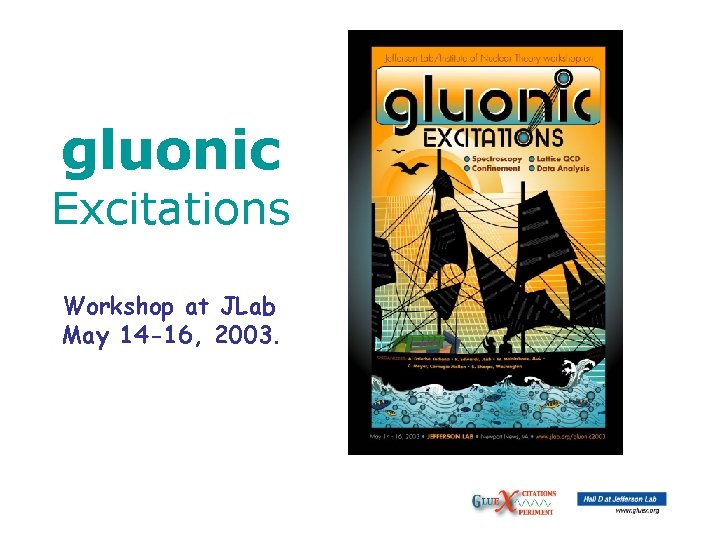
gluonic Excitations Workshop at JLab May 14 -16, 2003.
Nbca glue
What are glue words
Cyanoacrylate fuming fingerprints
Crazy glue
Second degree burn from hot glue gun
The glue group
Pan melt glue floral design
Aws glue gui
Glue record dns
Pva glue wiki
Structure of haemoglobin
Is riveting a permanent joint
Glue phobia
Glue a picture for each greeting below
Erd vs erm
The potion contained fruit biscuits and glue
Glue
Write five sentences about your family
Check glue records
Scissors and glue stick
Lignosulfonate glue price
Aws batch icon
Pwa glue
Các môn thể thao bắt đầu bằng tiếng chạy
Chó sói
Thiếu nhi thế giới liên hoan
Sự nuôi và dạy con của hổ
điện thế nghỉ
Một số thể thơ truyền thống
Trời xanh đây là của chúng ta thể thơ
Ng-html
Các số nguyên tố là gì
Vẽ hình chiếu vuông góc của vật thể sau
Các châu lục và đại dương trên thế giới
Thế nào là hệ số cao nhất
Tư thế worms-breton
ưu thế lai là gì
Hệ hô hấp
Tư thế ngồi viết
đặc điểm cơ thể của người tối cổ
Cái miệng bé xinh thế chỉ nói điều hay thôi
Mật thư tọa độ 5x5
Bổ thể
Tư thế ngồi viết
Thẻ vin
Thể thơ truyền thống
Hát lên người ơi alleluia
Các châu lục và đại dương trên thế giới
Từ ngữ thể hiện lòng nhân hậu
Diễn thế sinh thái là
Vẽ hình chiếu vuông góc của vật thể sau
V cc
Phép trừ bù
Tỉ lệ cơ thể trẻ em
Sự nuôi và dạy con của hổ
Lời thề hippocrates
đại từ thay thế
Quá trình desamine hóa có thể tạo ra
Cong thức tính động năng
Hình ảnh bộ gõ cơ thể búng tay
Thế nào là mạng điện lắp đặt kiểu nổi
Các loại đột biến cấu trúc nhiễm sắc thể
Biện pháp chống mỏi cơ
Vẽ hình chiếu đứng bằng cạnh của vật thể
độ dài liên kết
Marty lobdell study less study smart
Ecological study vs cohort study