PHY 110 Introduction to Physics Dr Henry SC
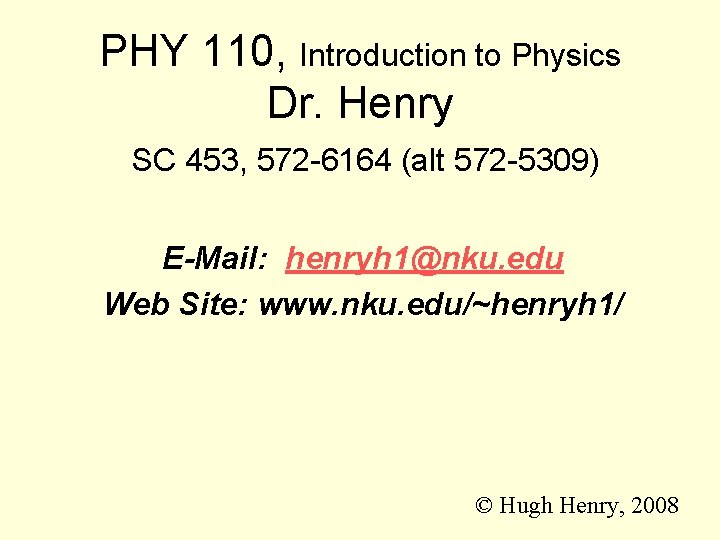
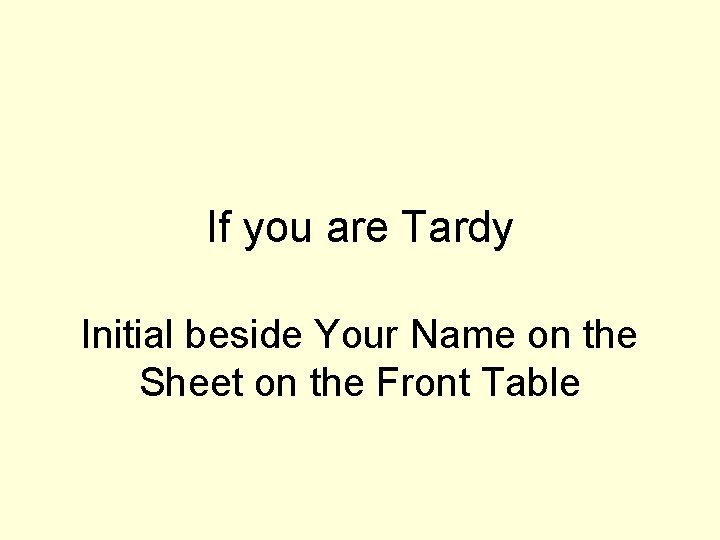
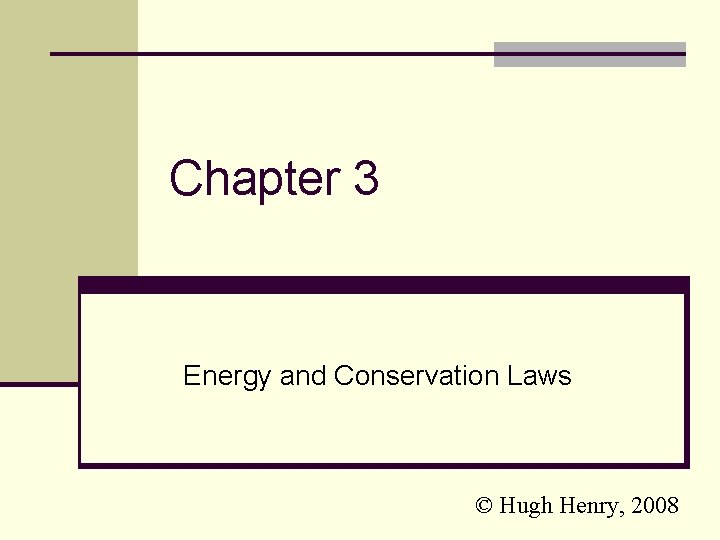
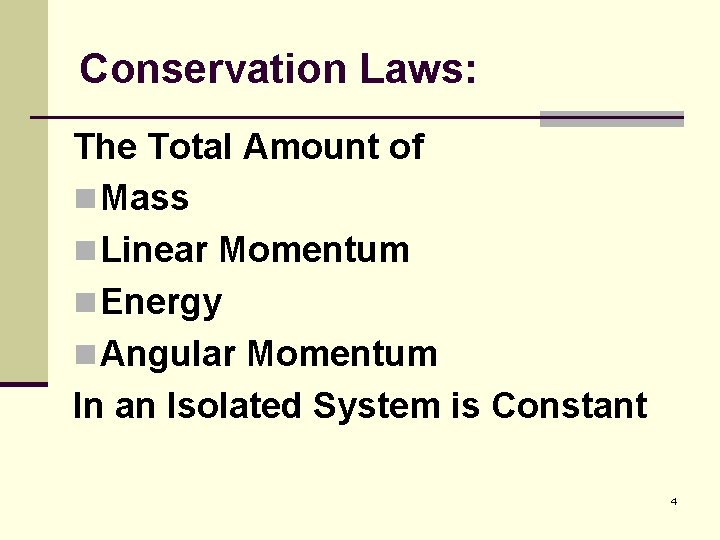
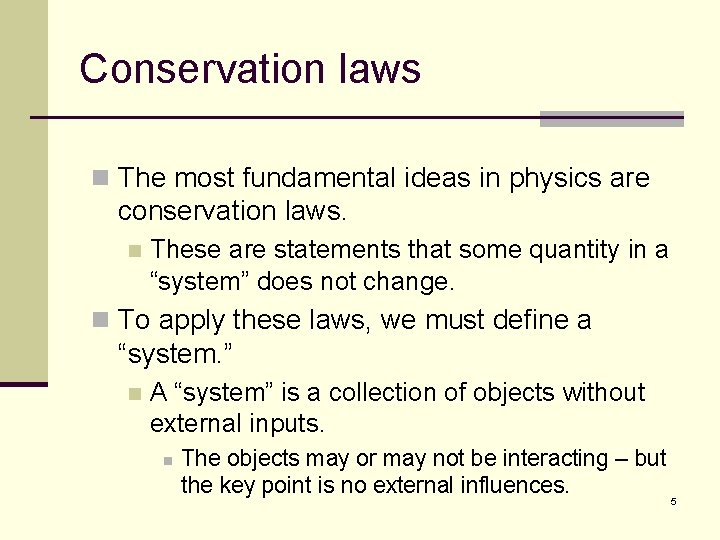
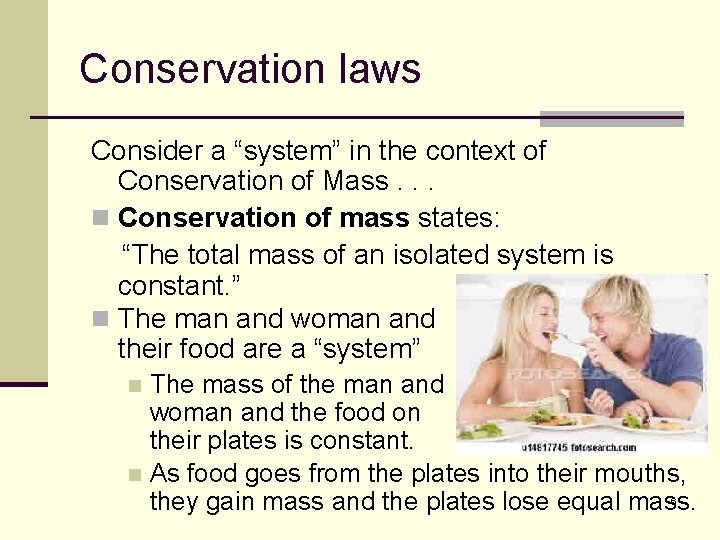
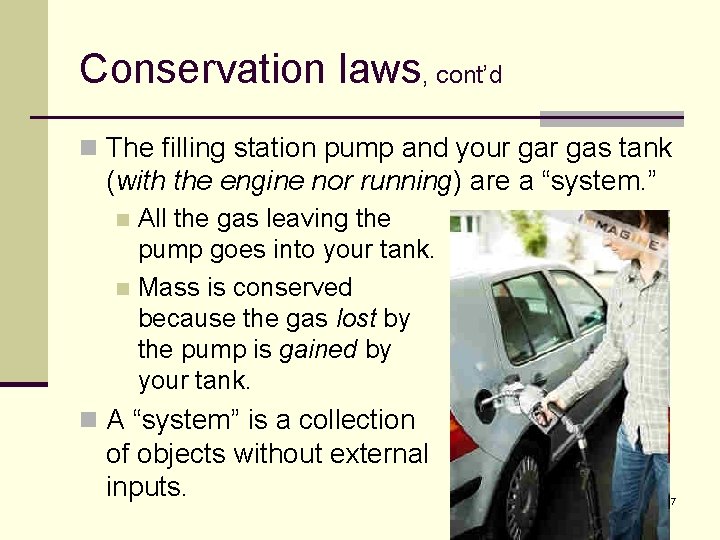
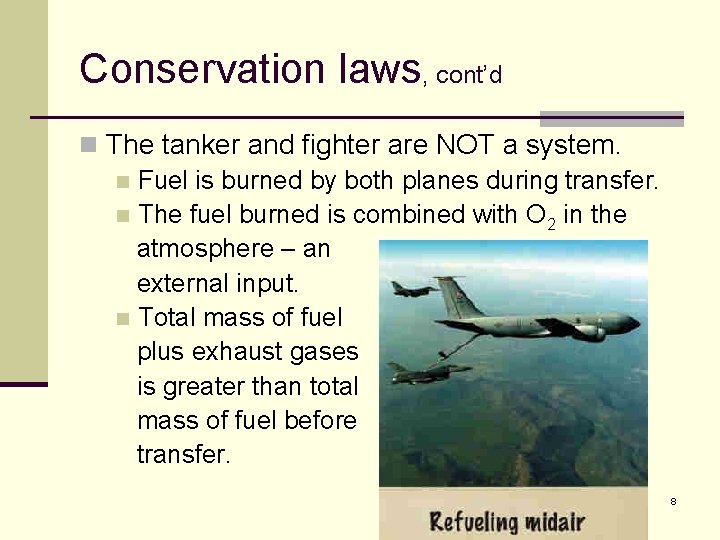
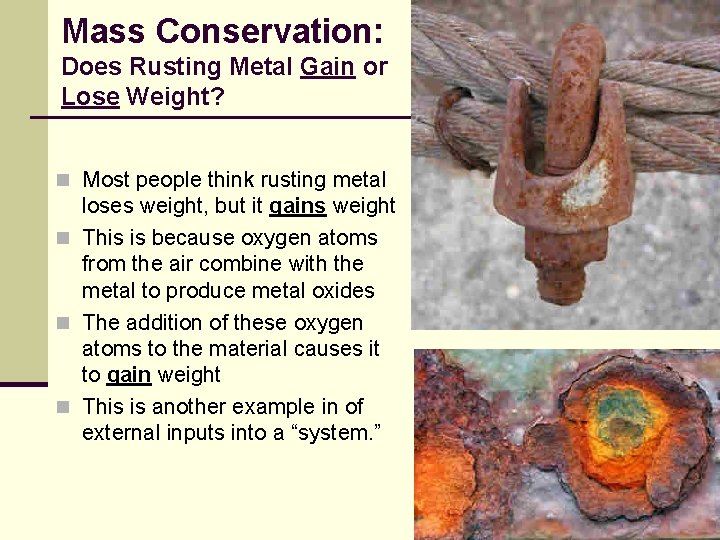
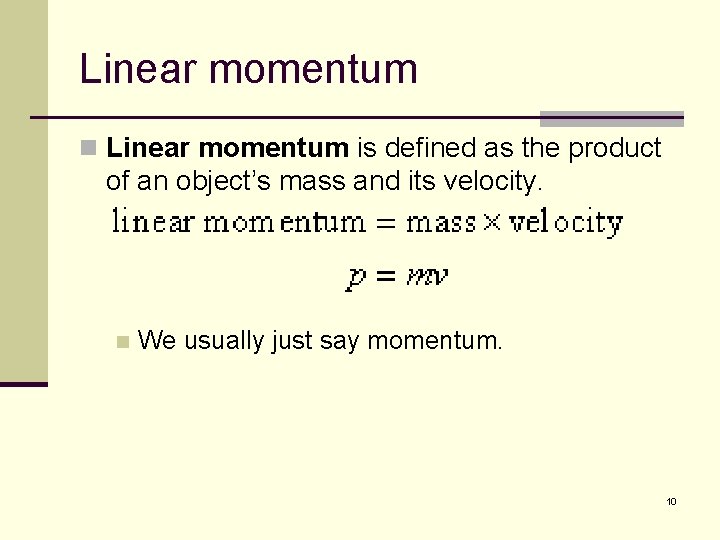
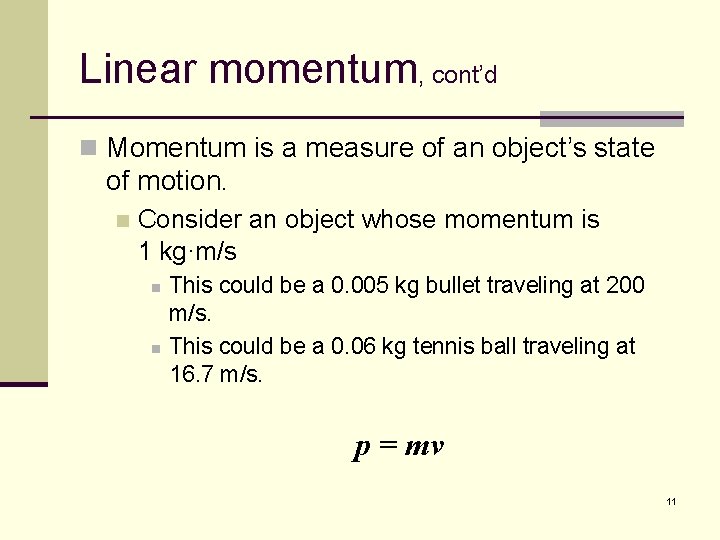
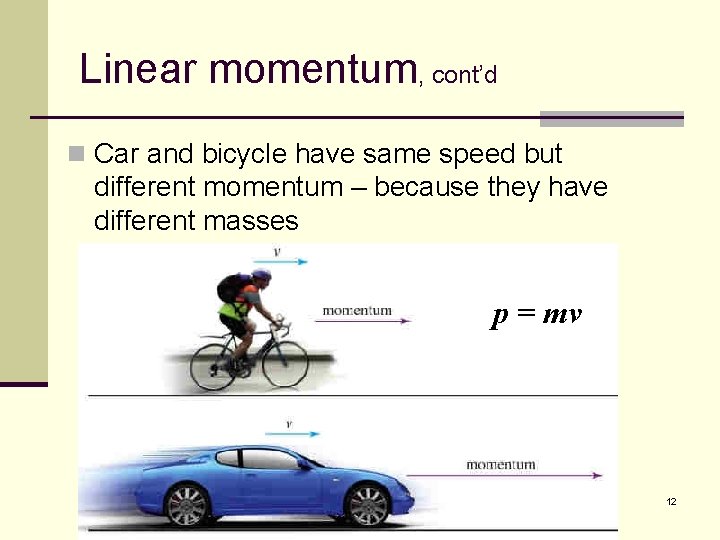
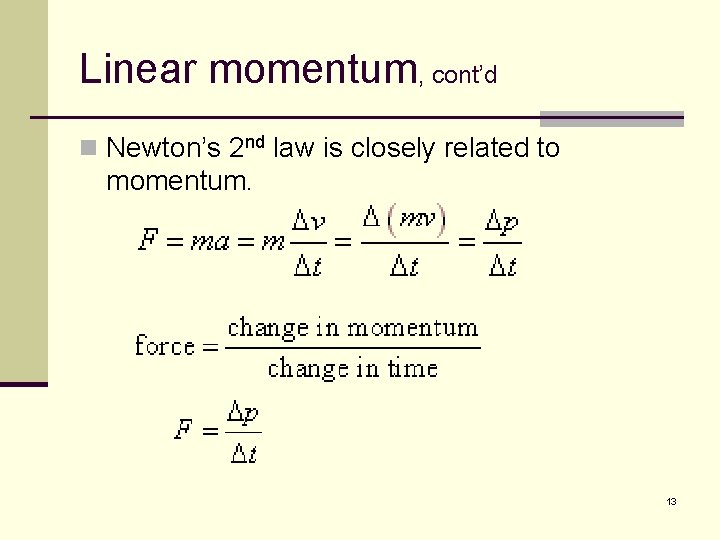
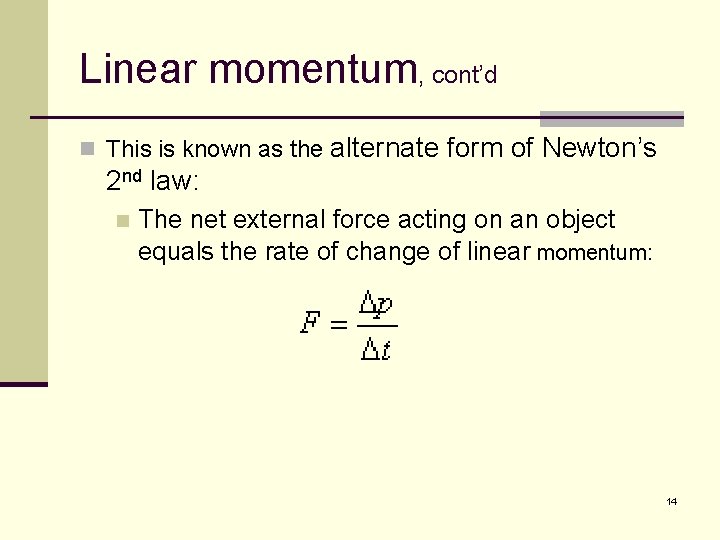
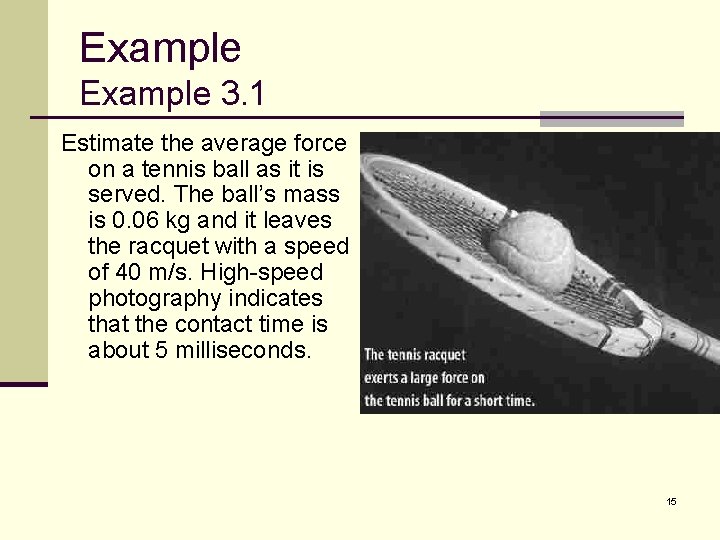
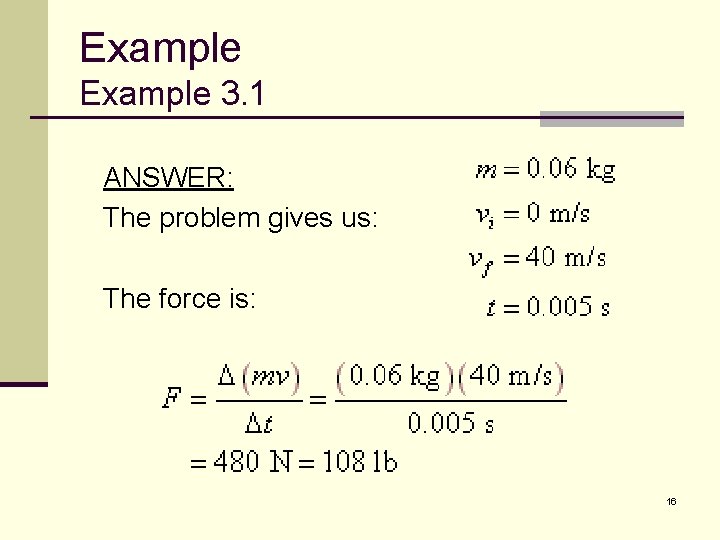
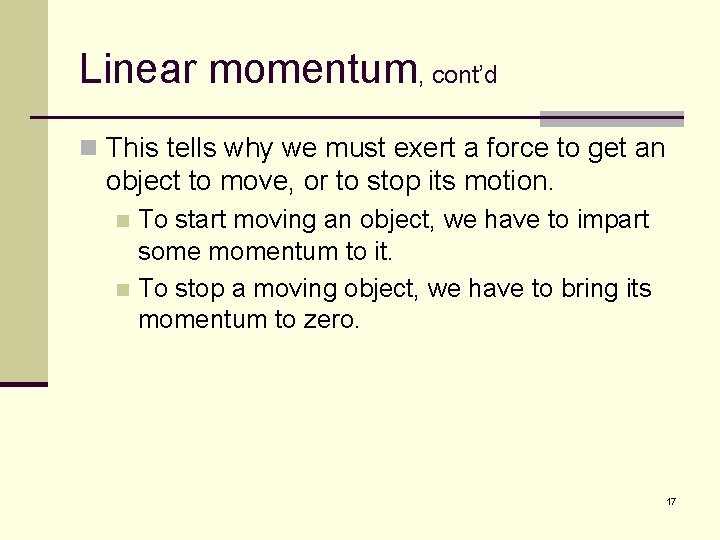
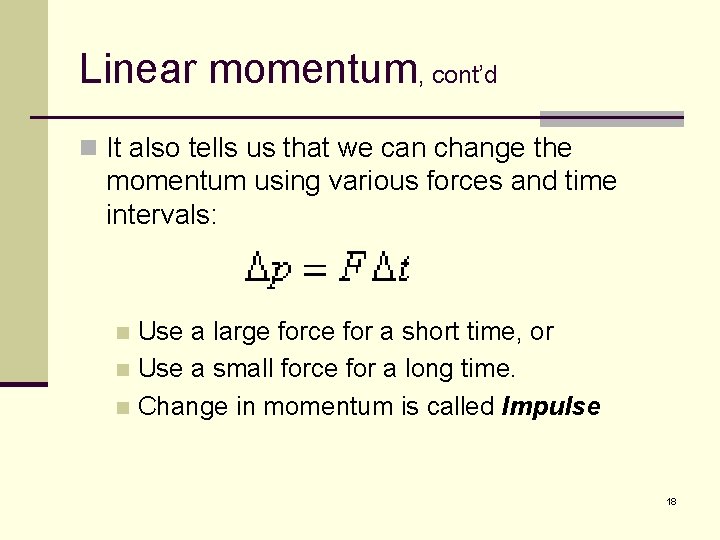
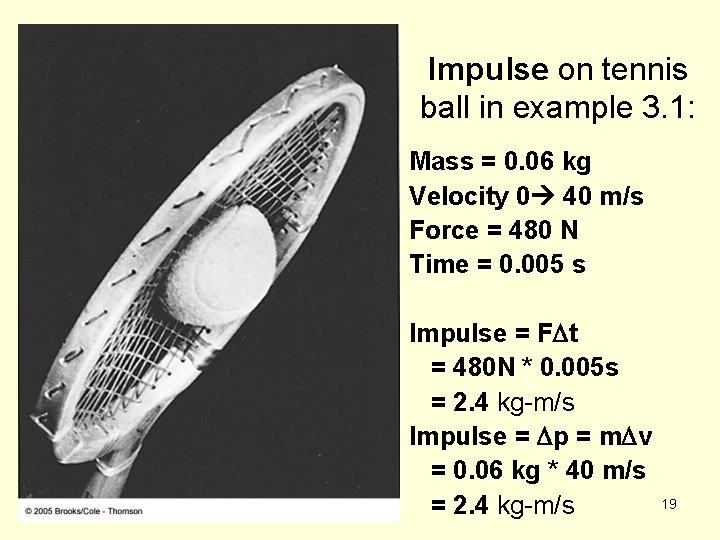
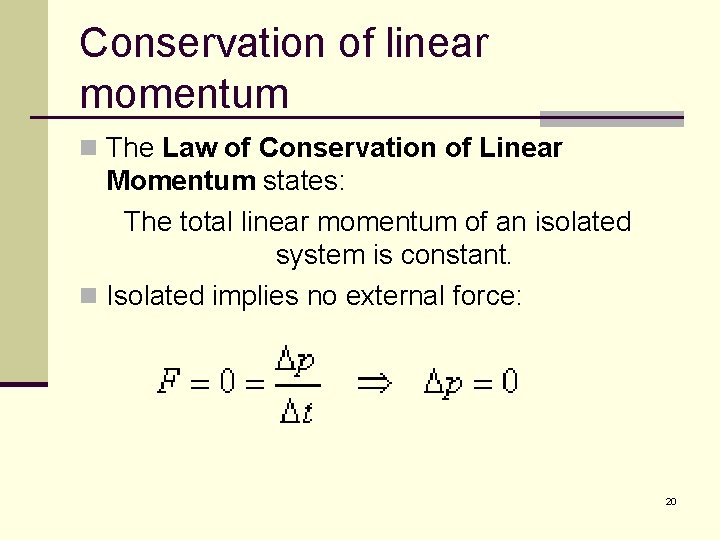
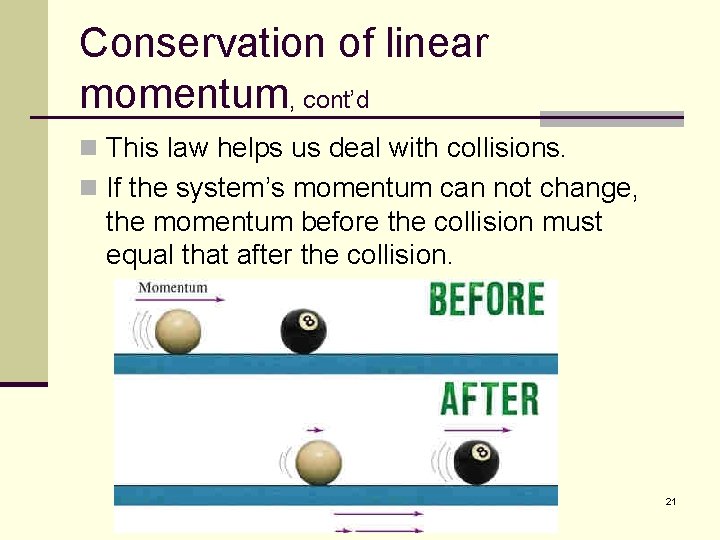
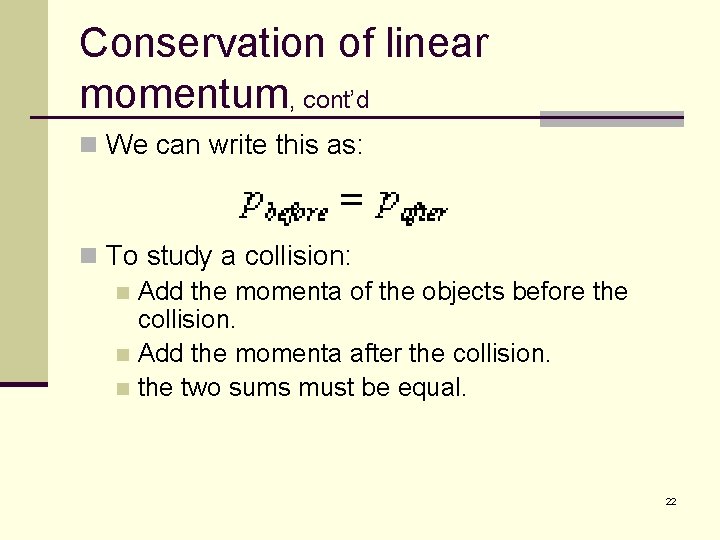
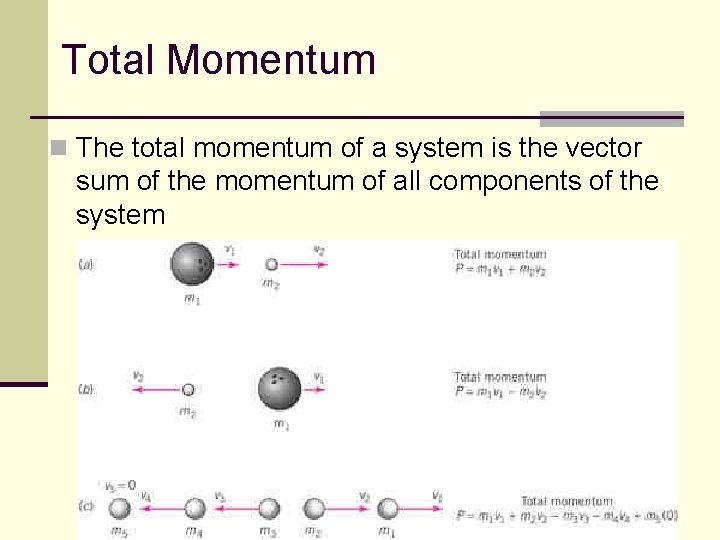
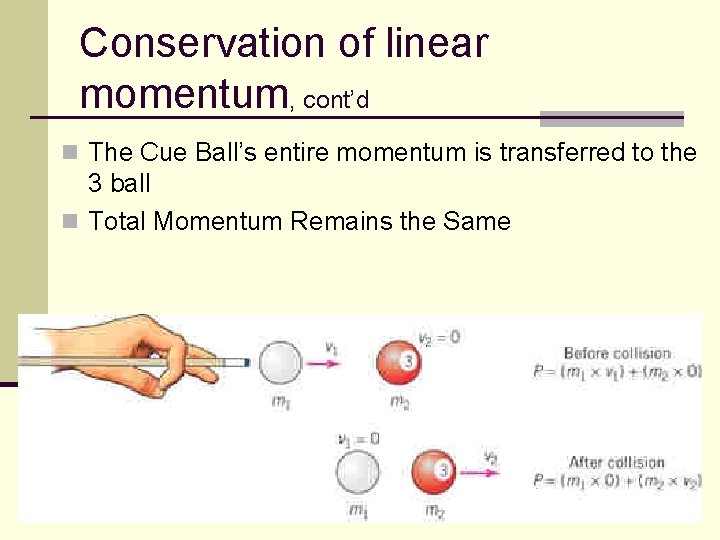
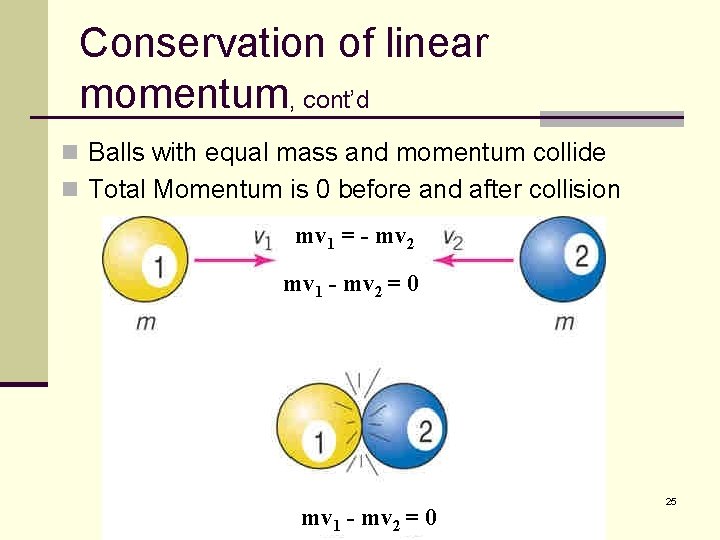
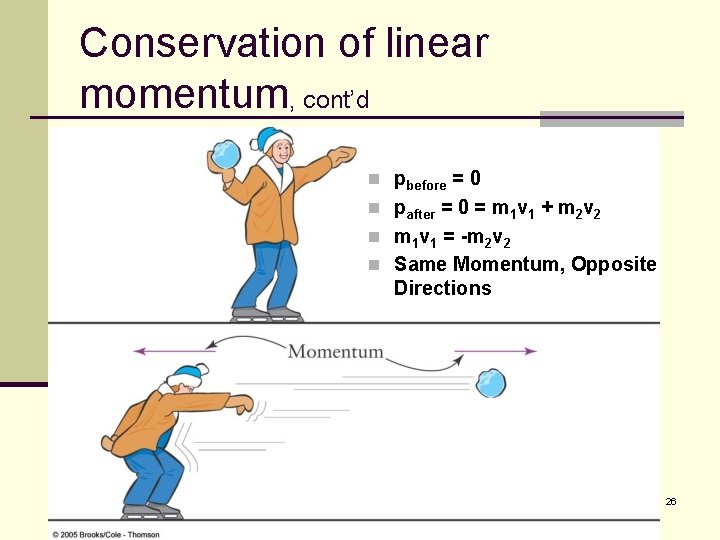
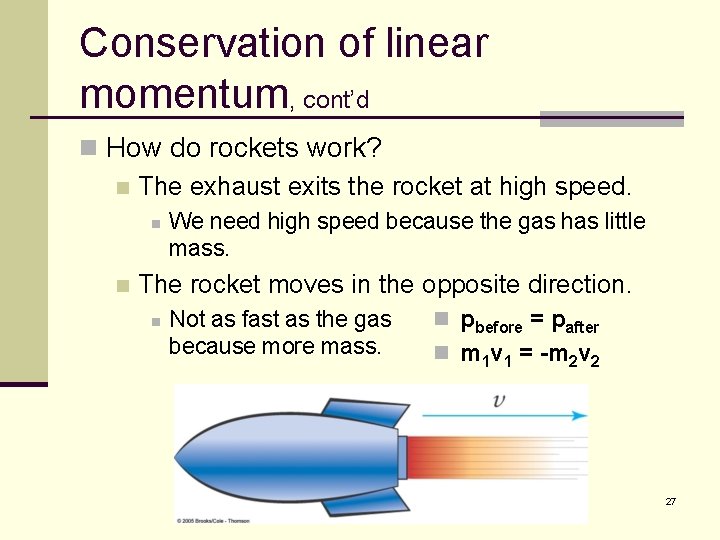
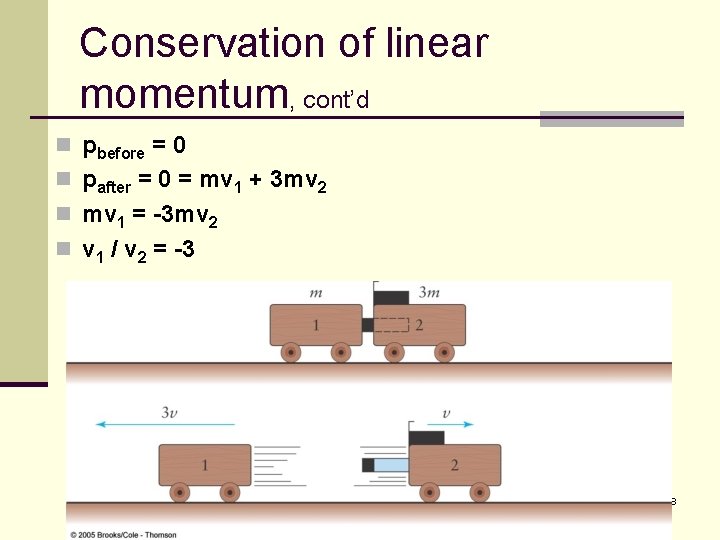
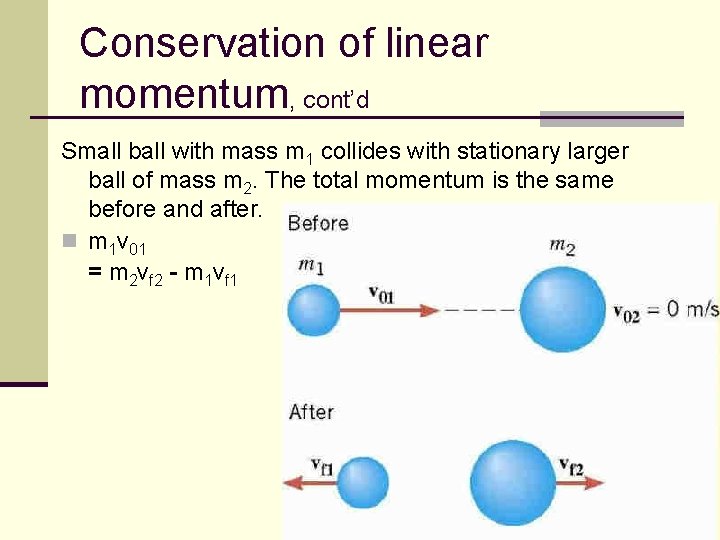
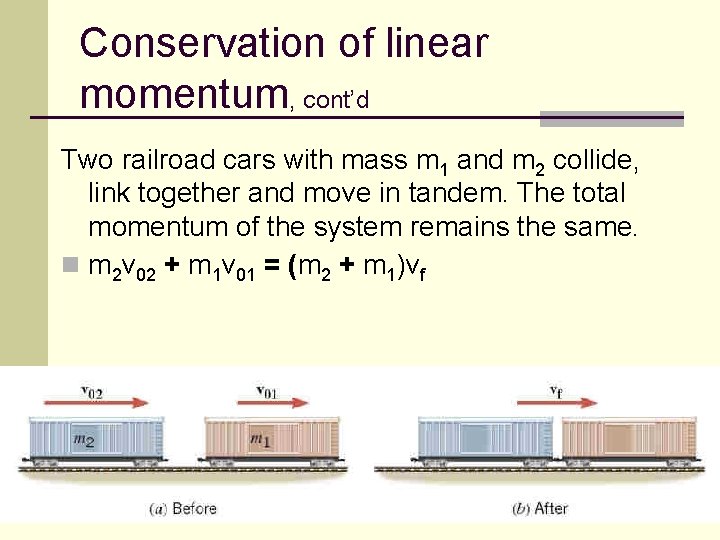
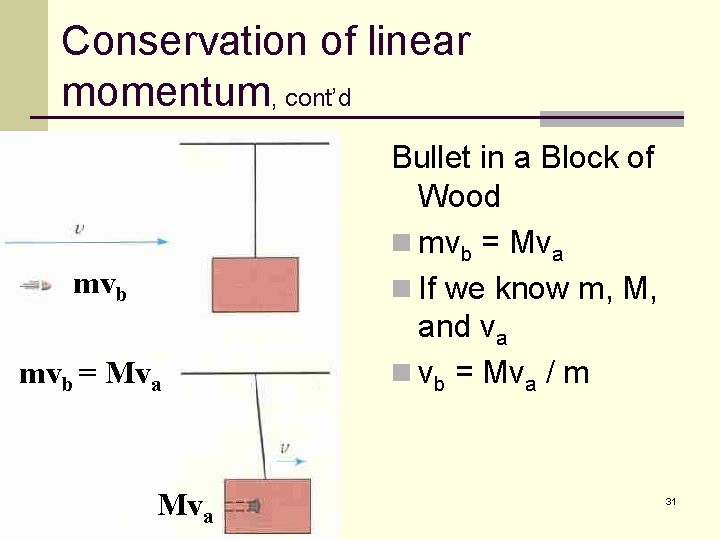
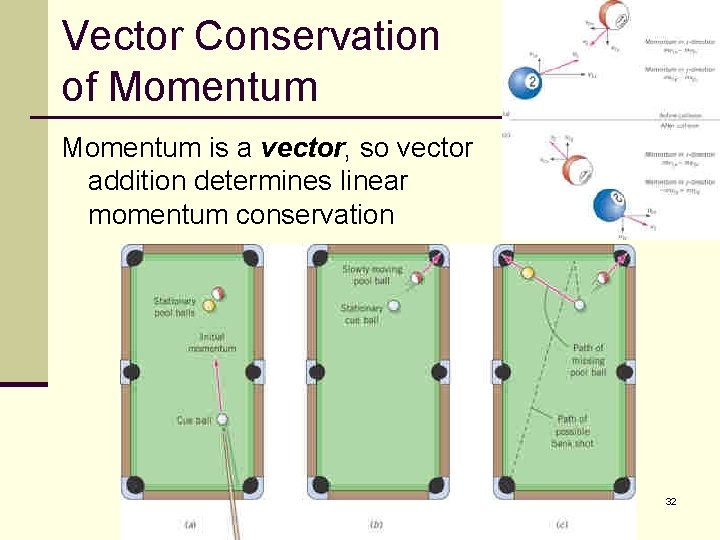
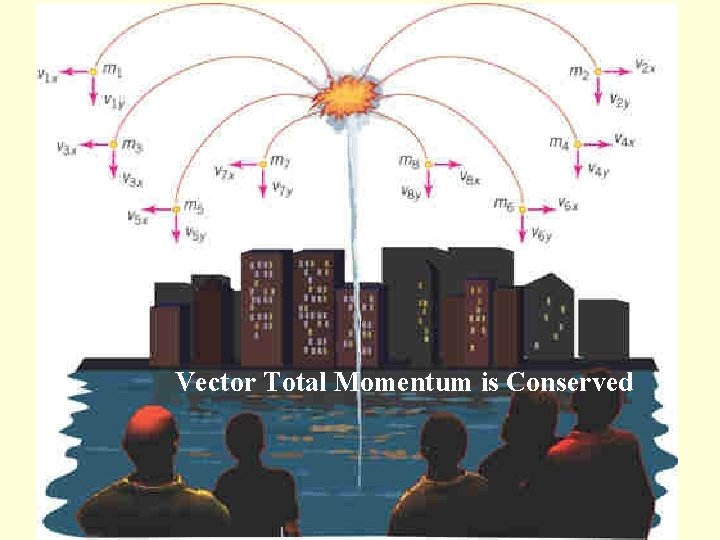
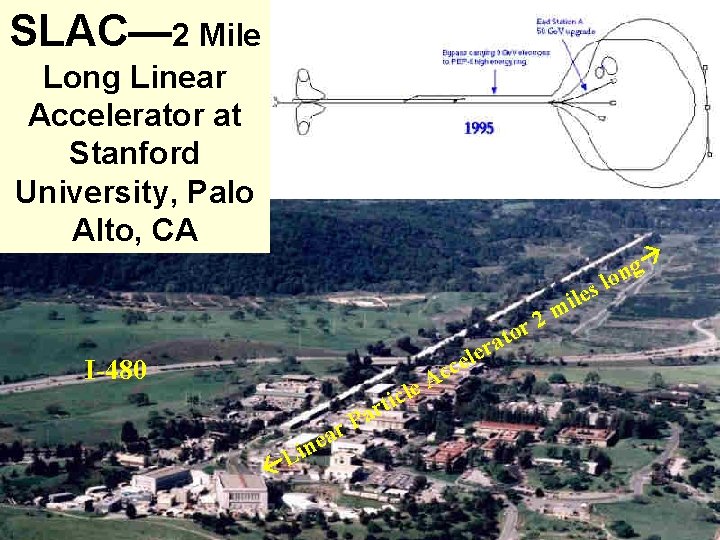
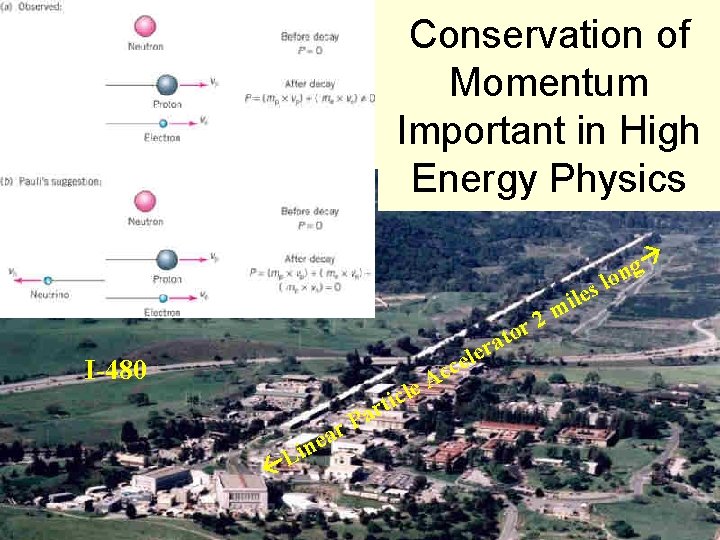
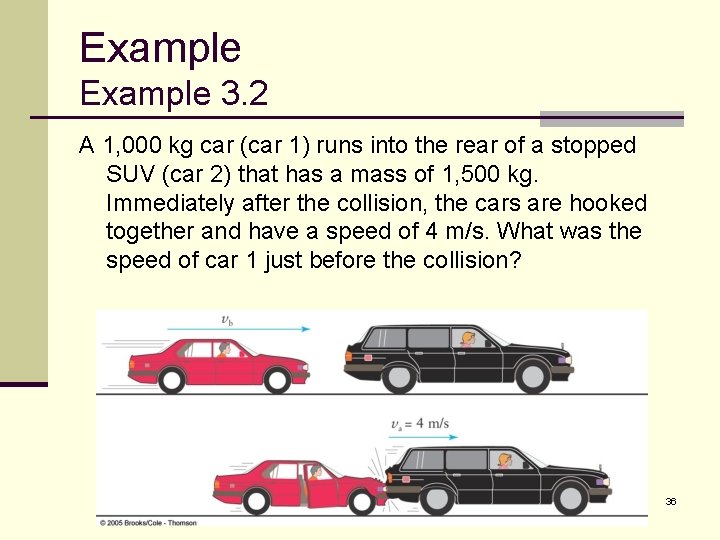
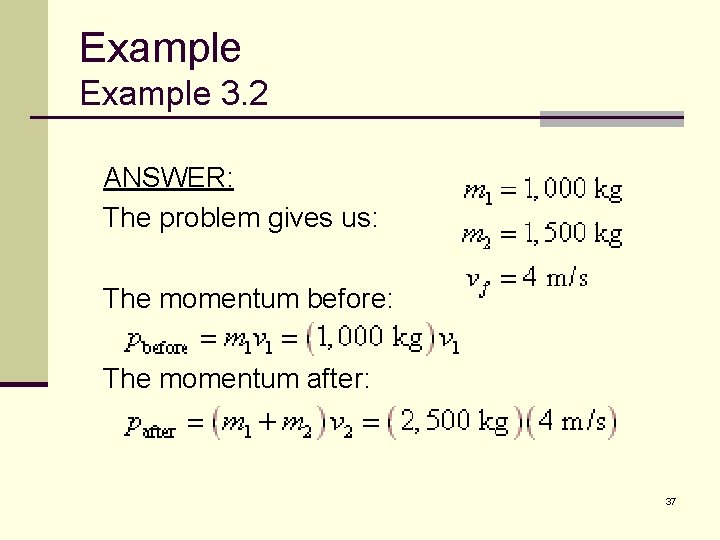
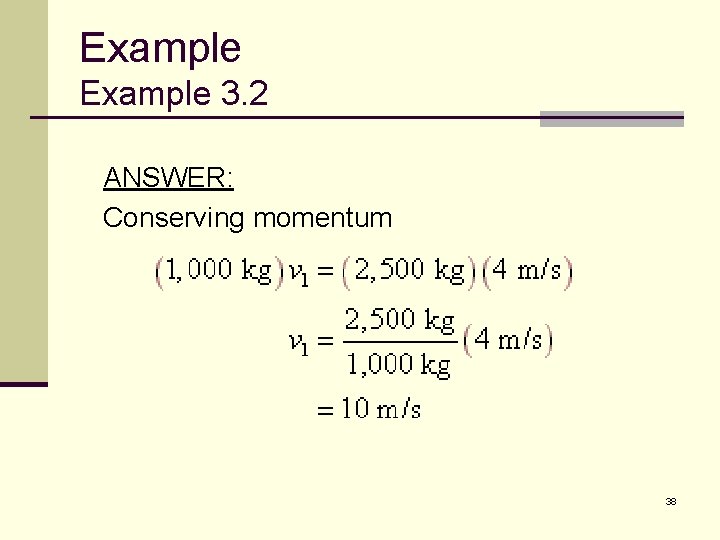
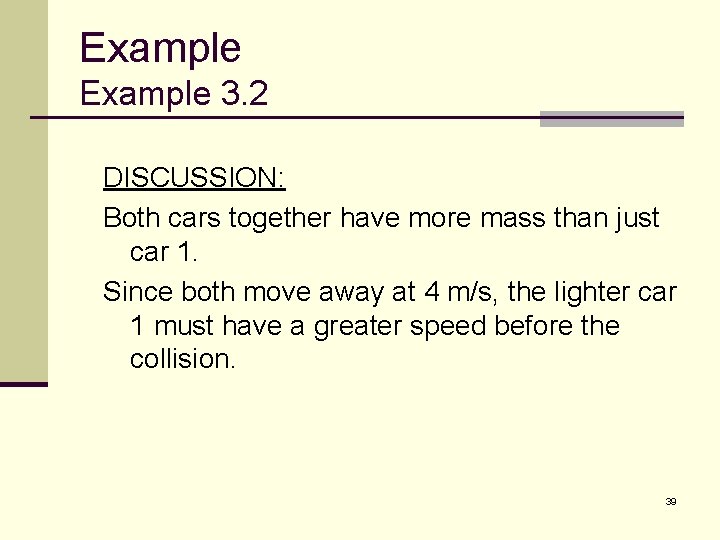
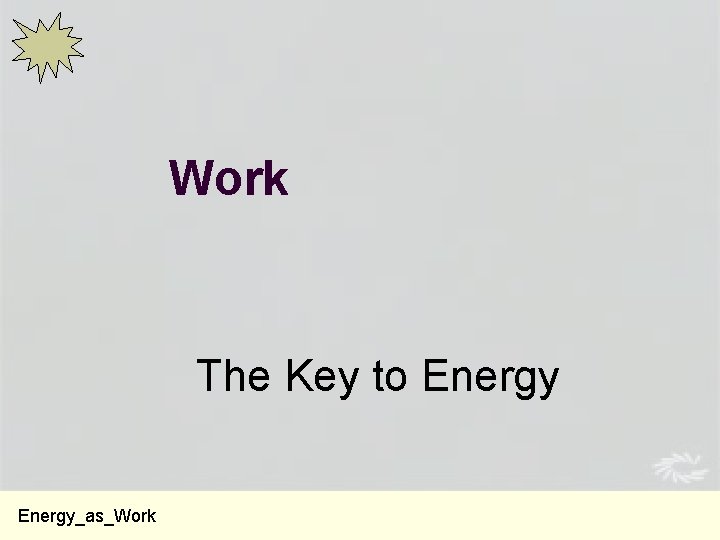
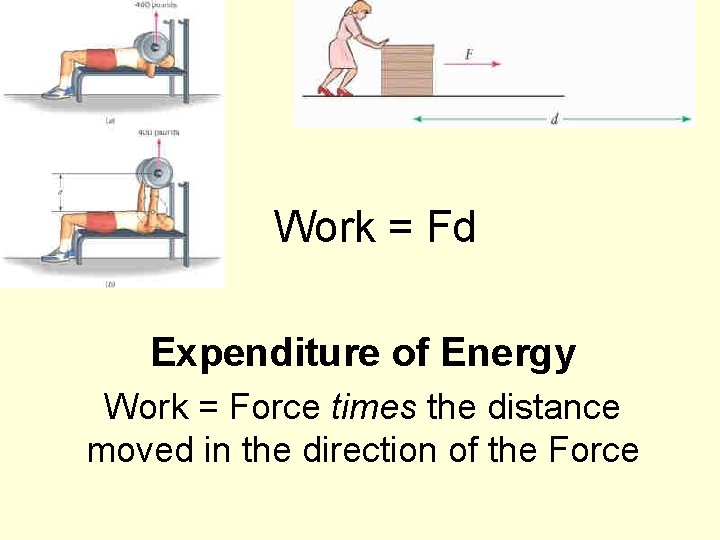
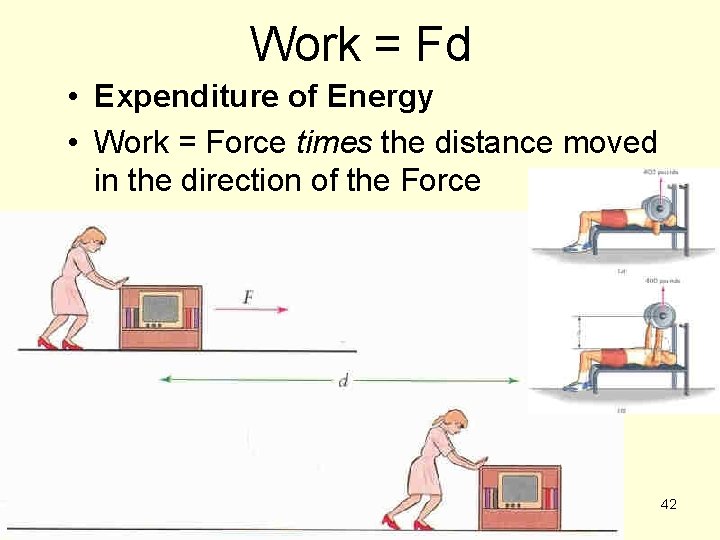
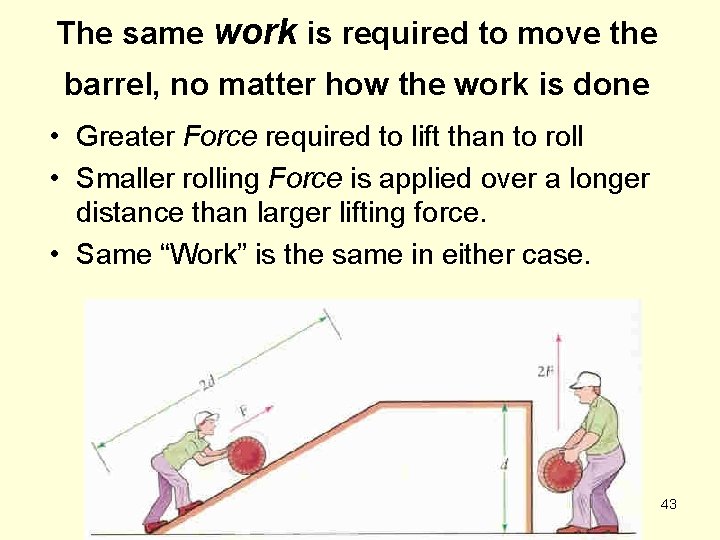
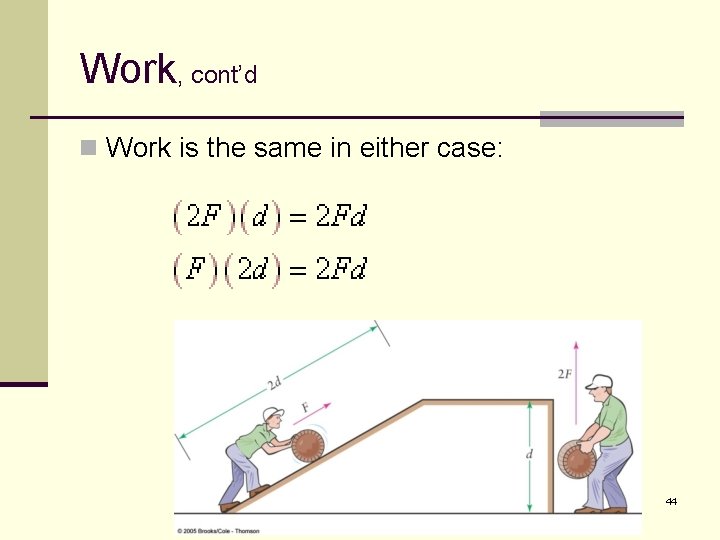
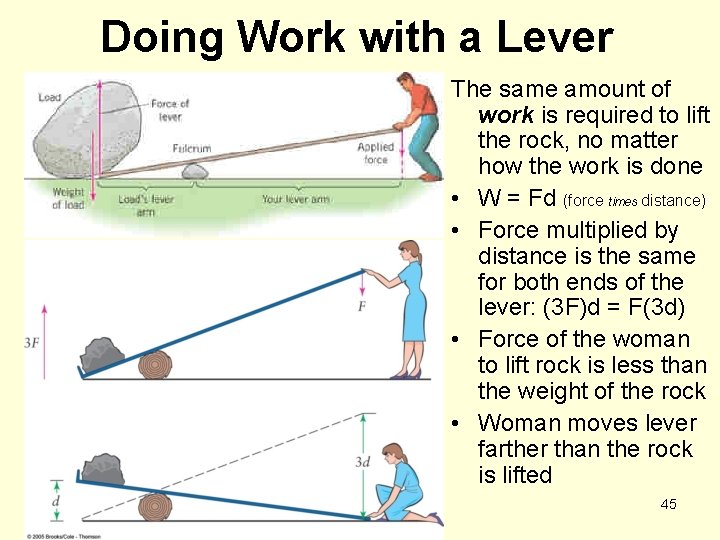
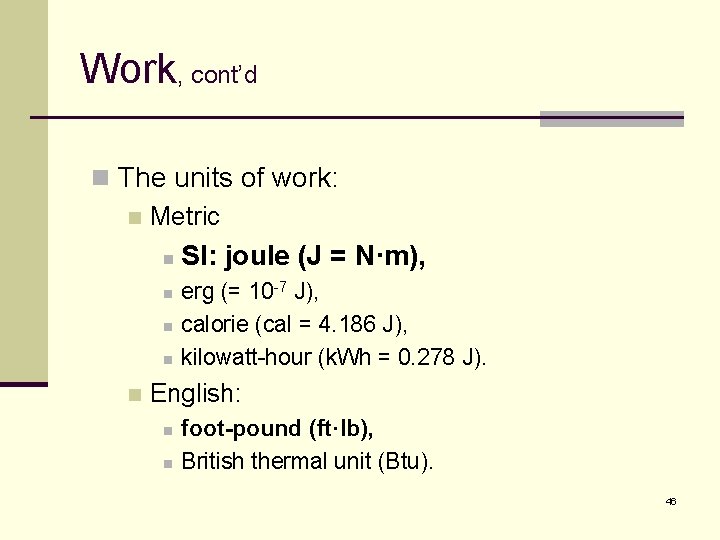
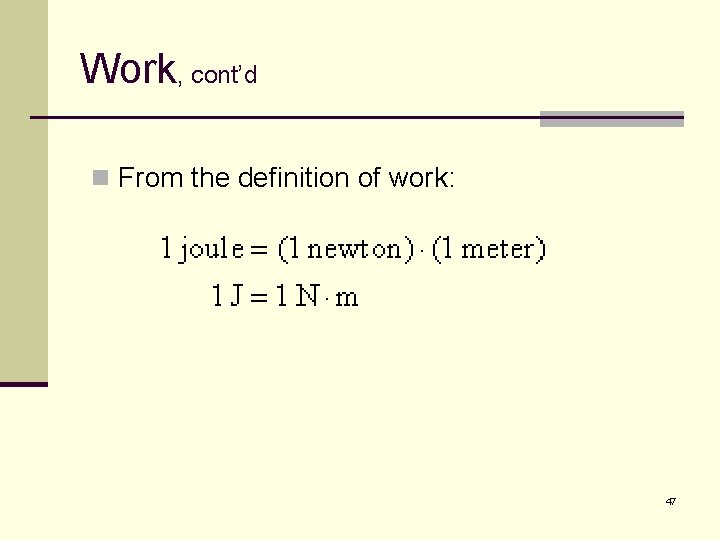
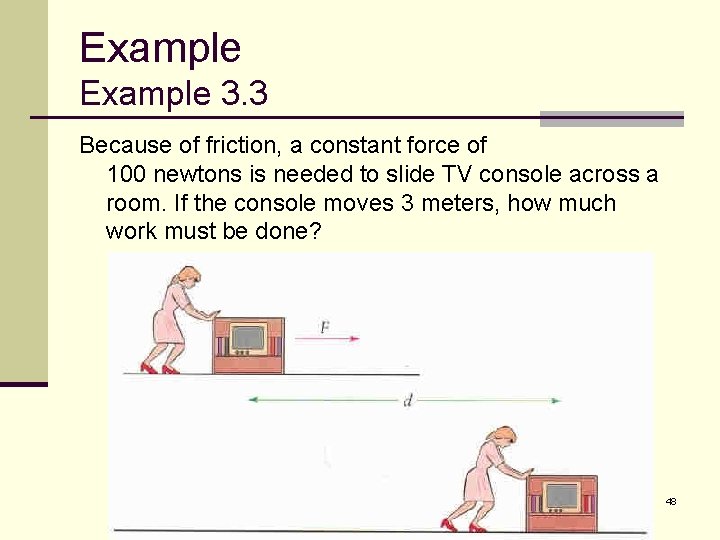
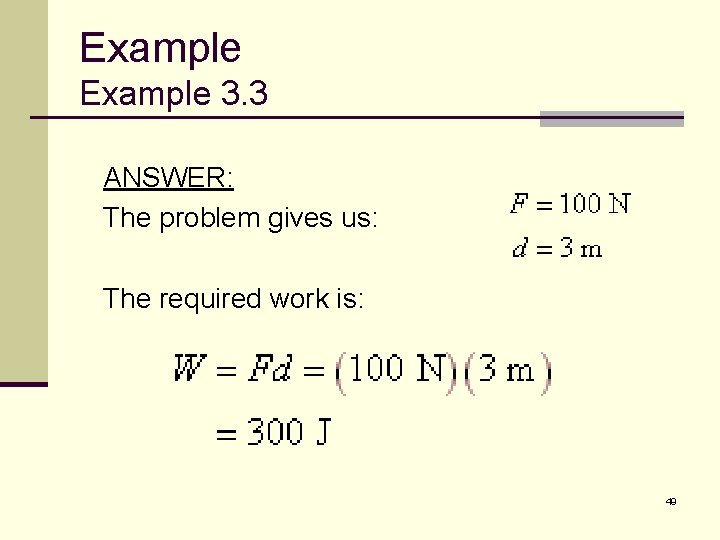
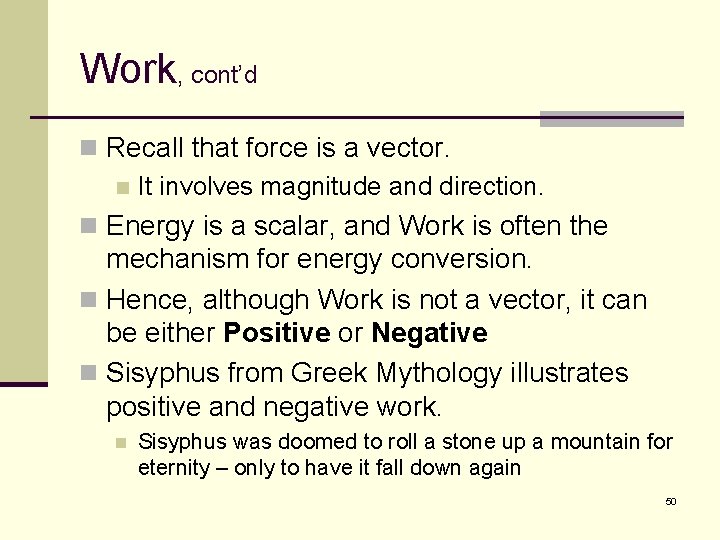
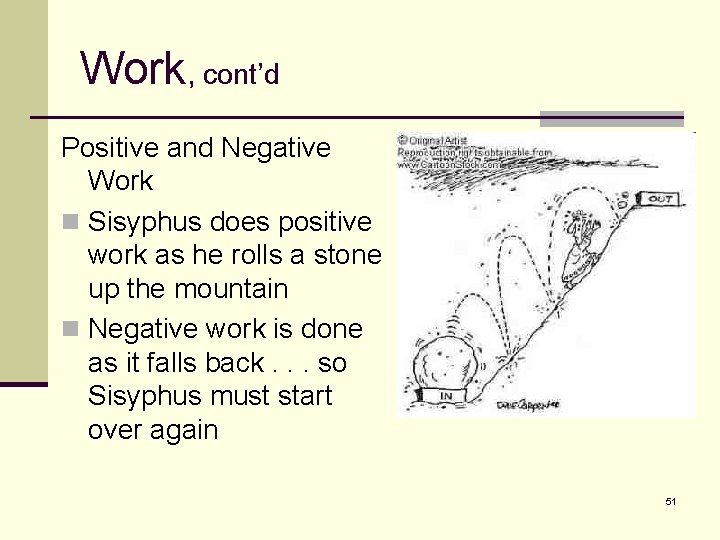
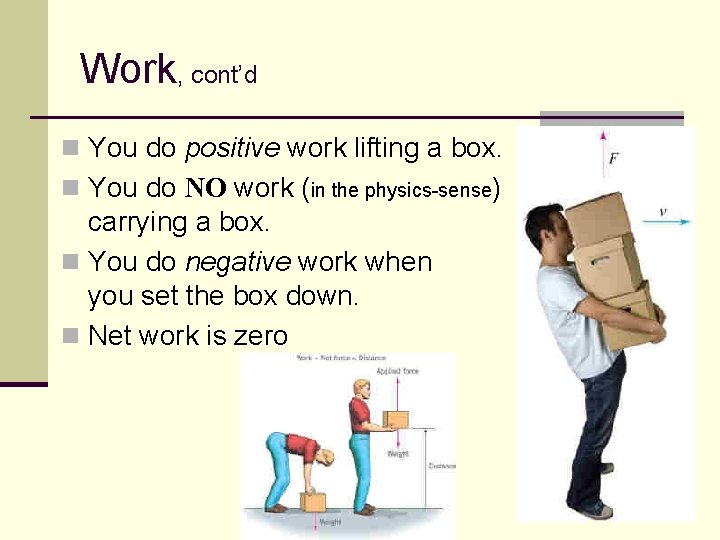
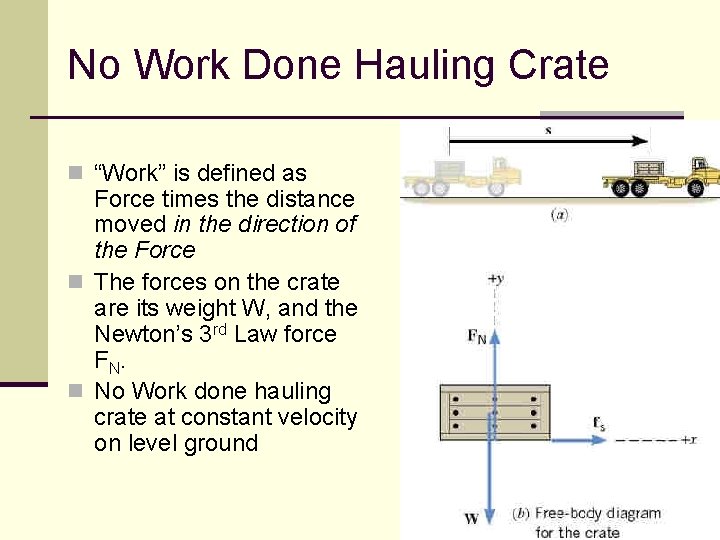
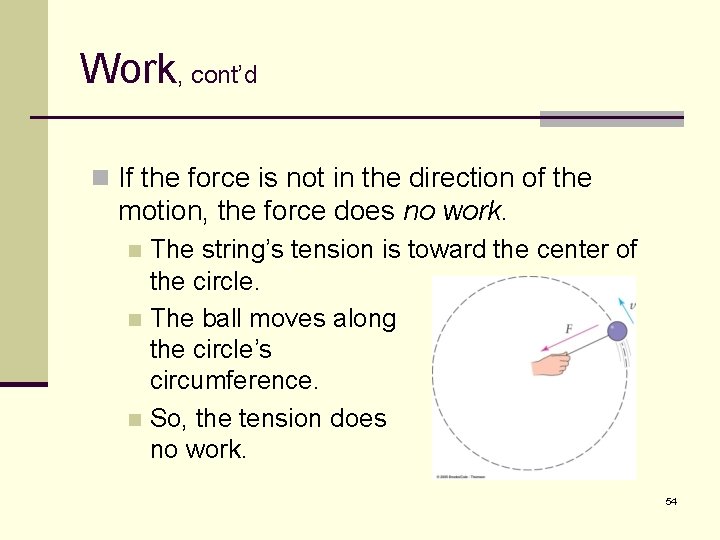
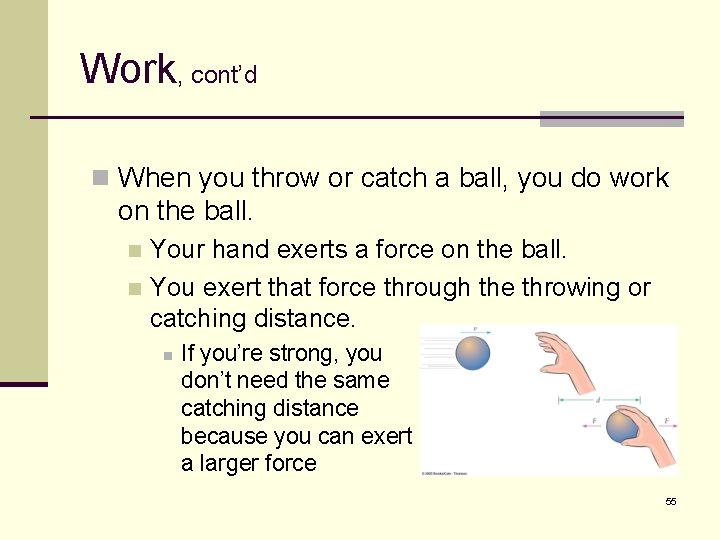
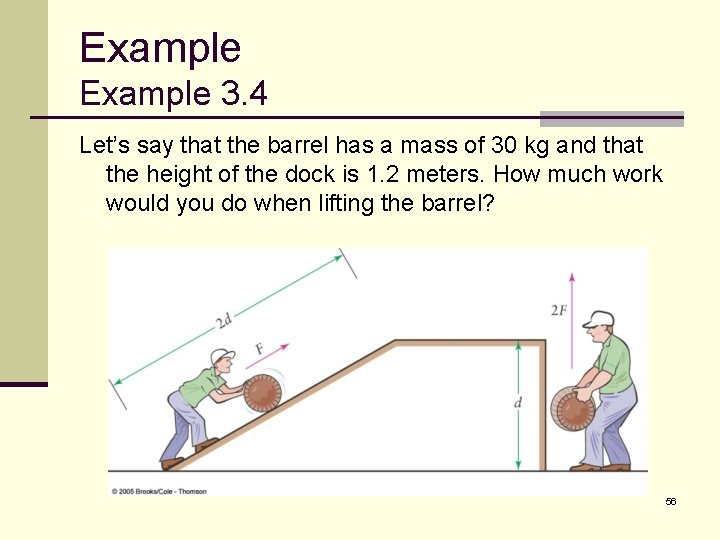
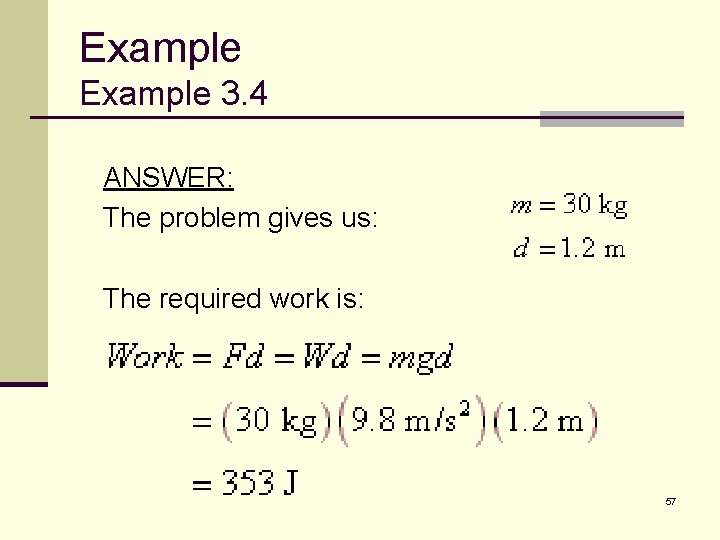
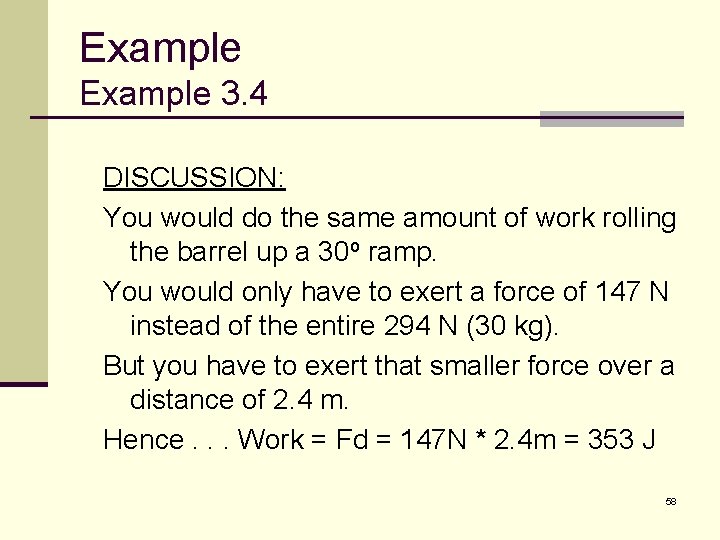
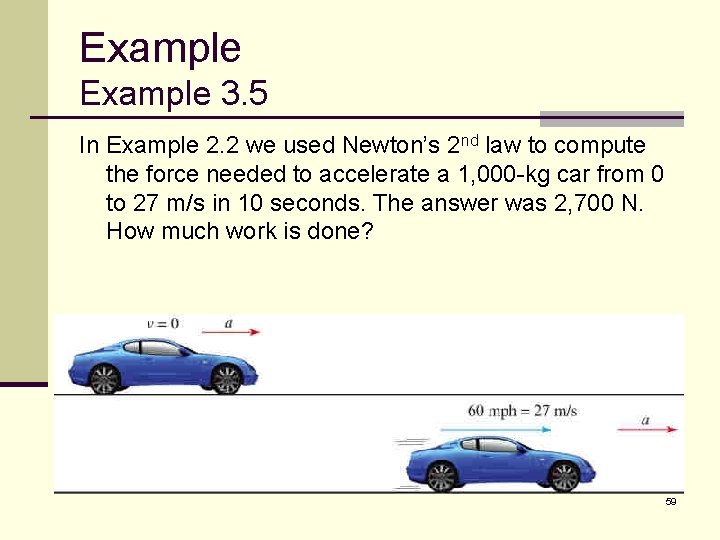
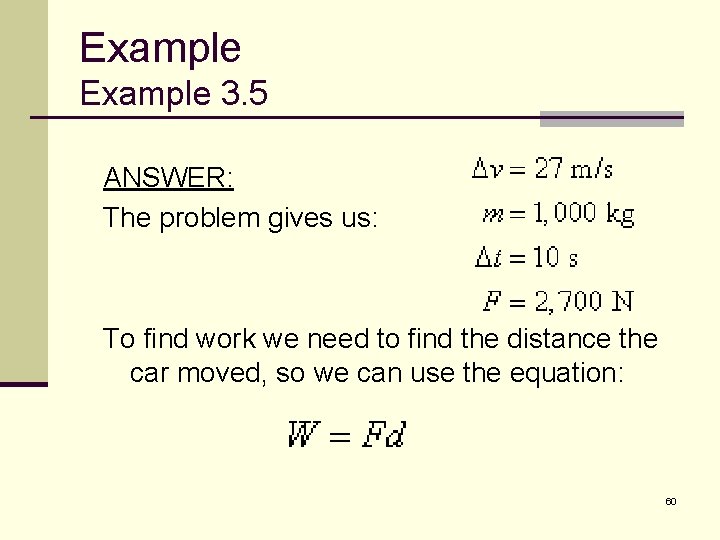
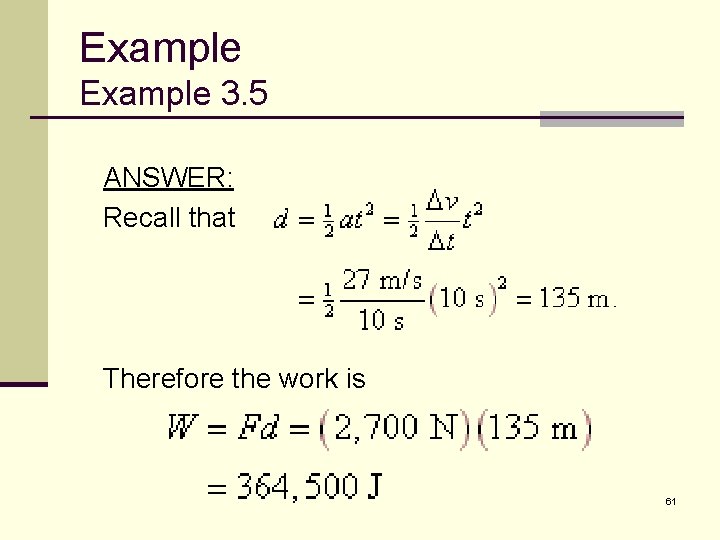
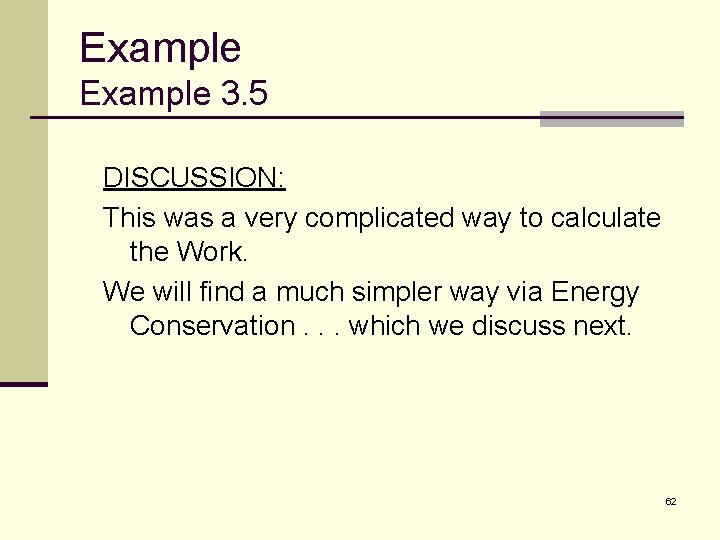
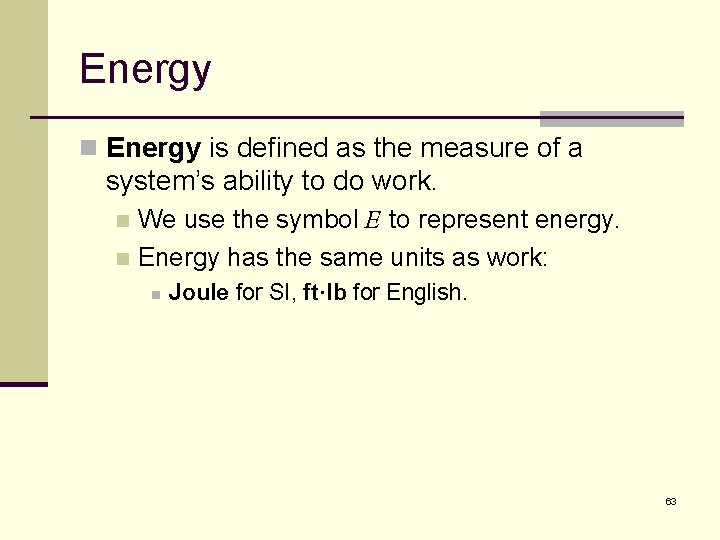
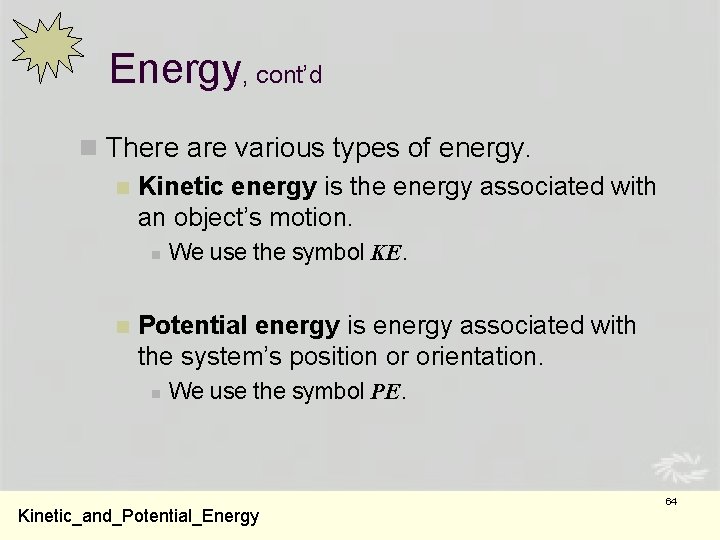
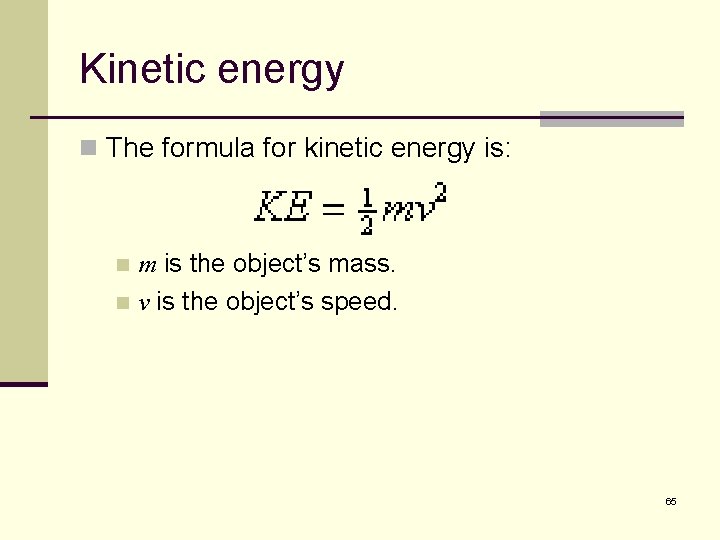
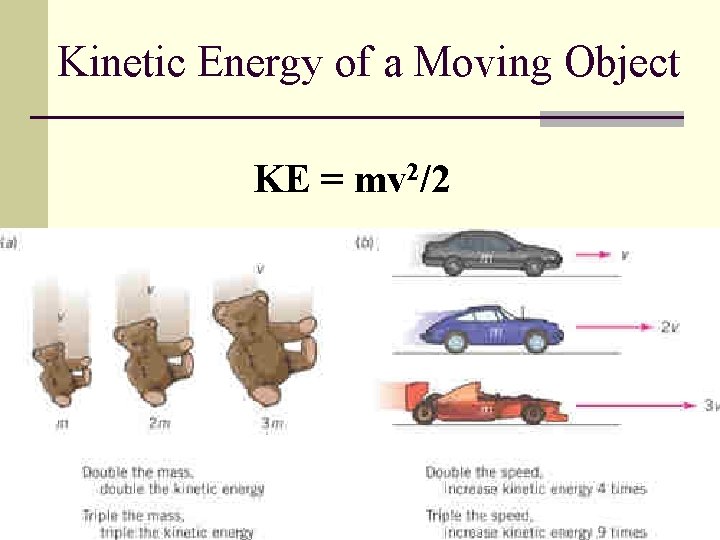
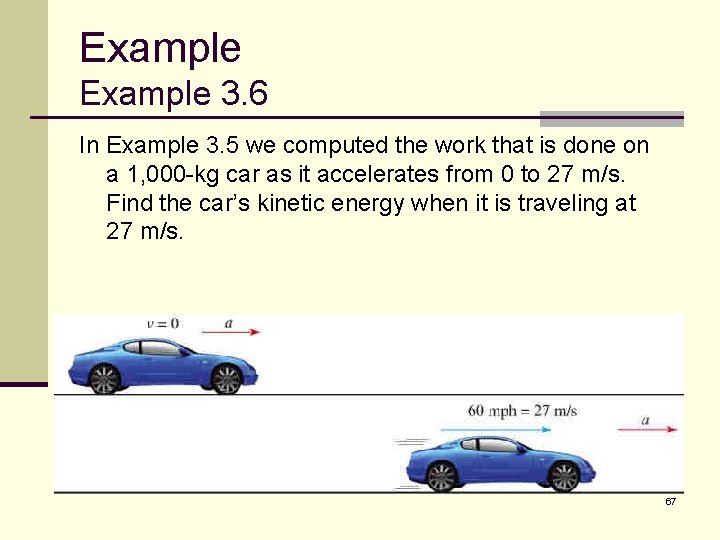
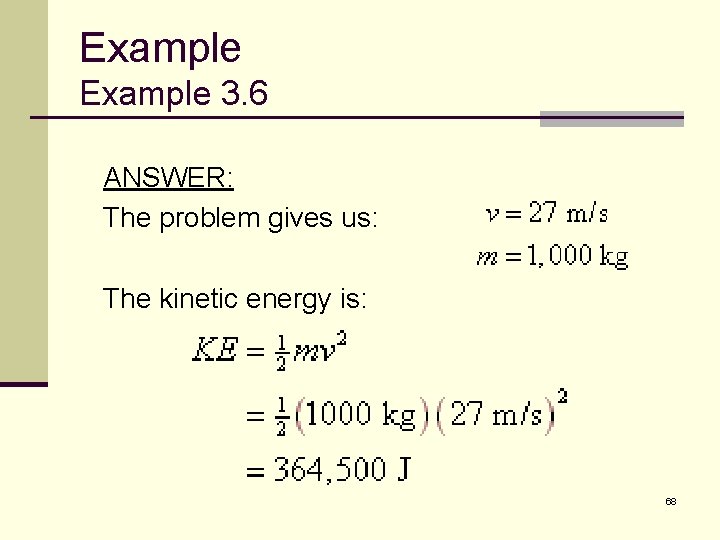
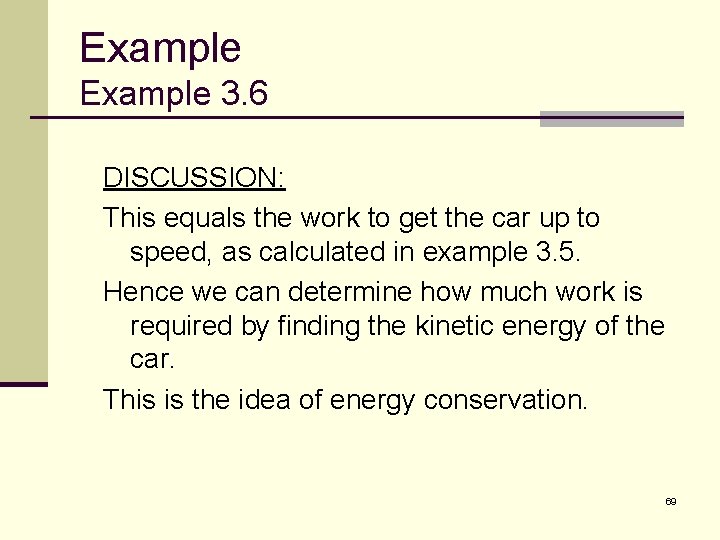
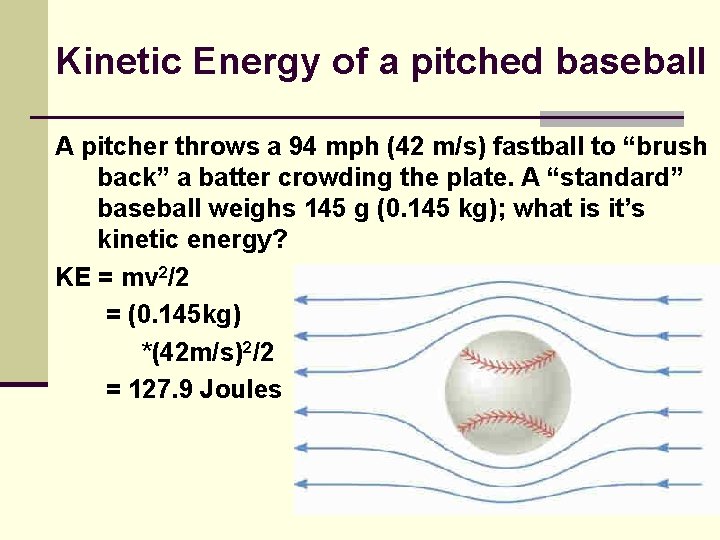
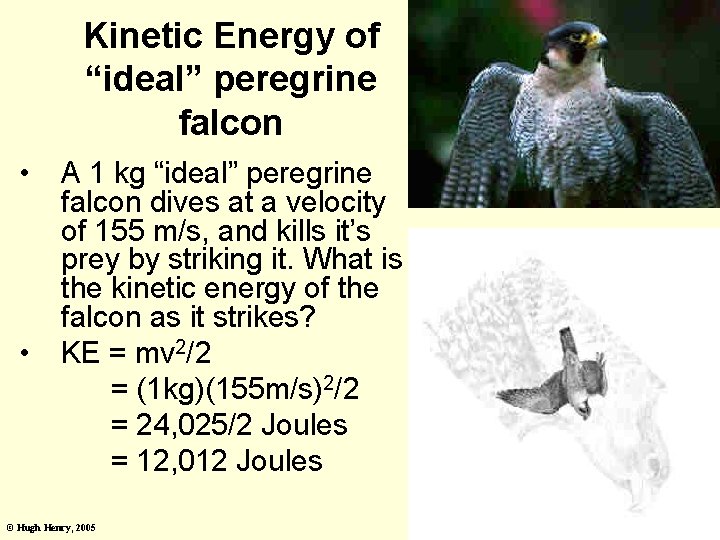
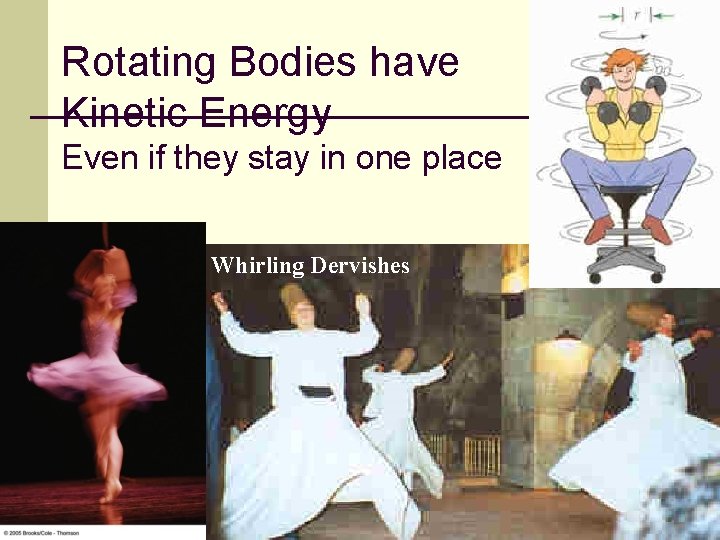
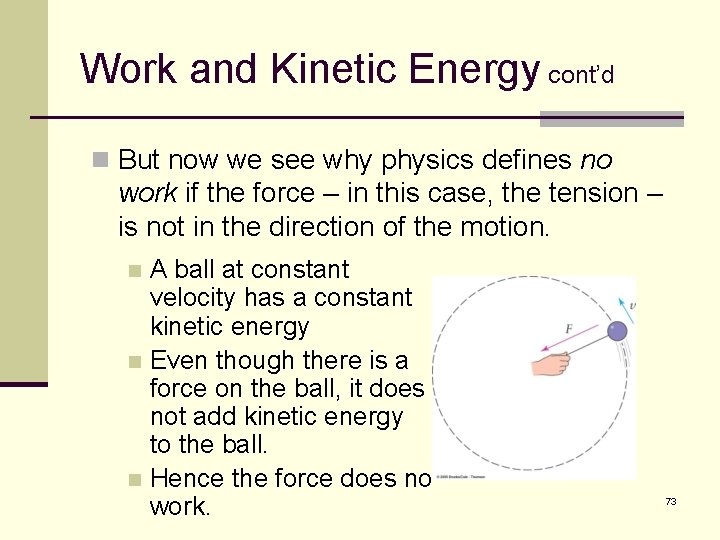
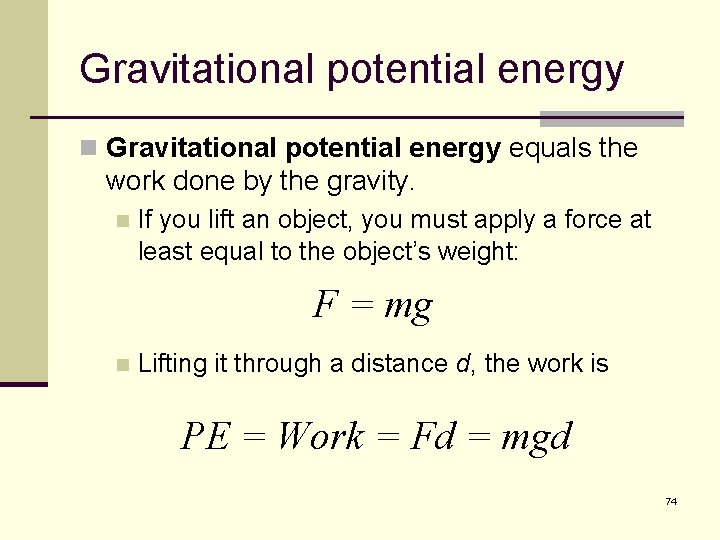
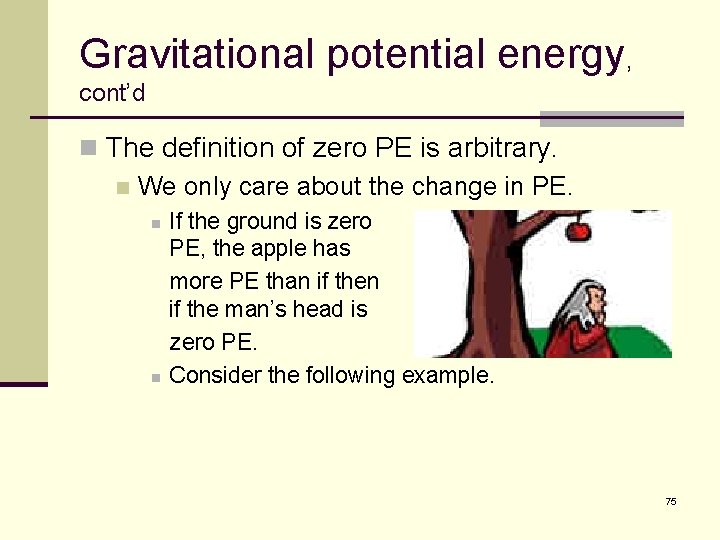
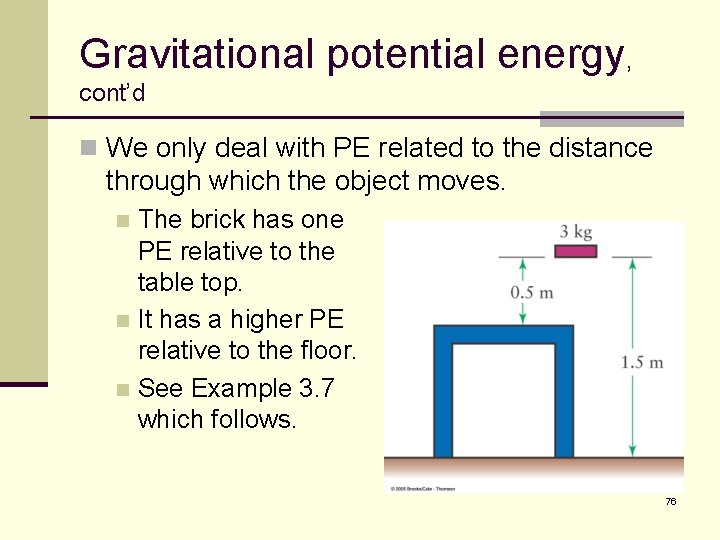
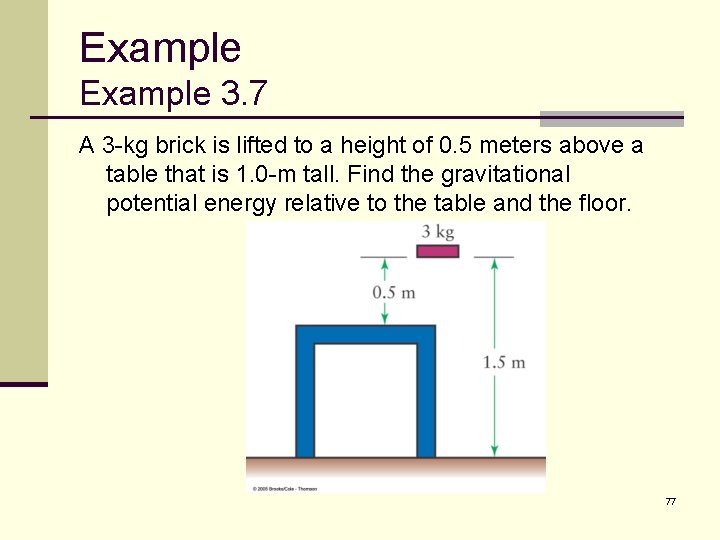
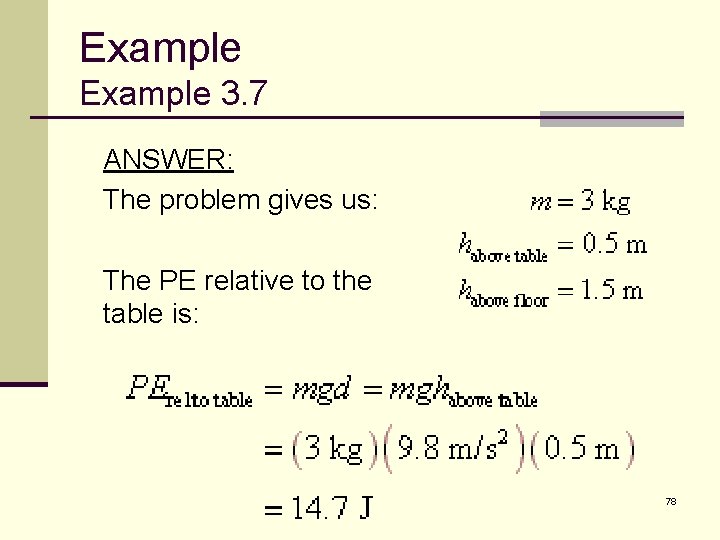
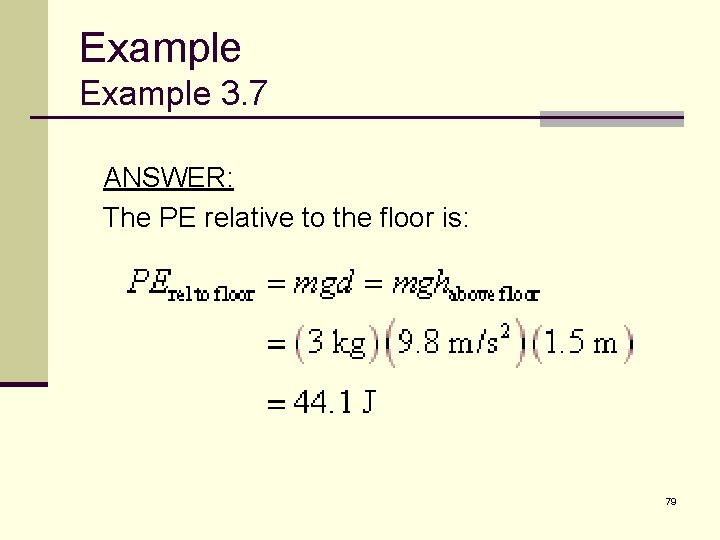
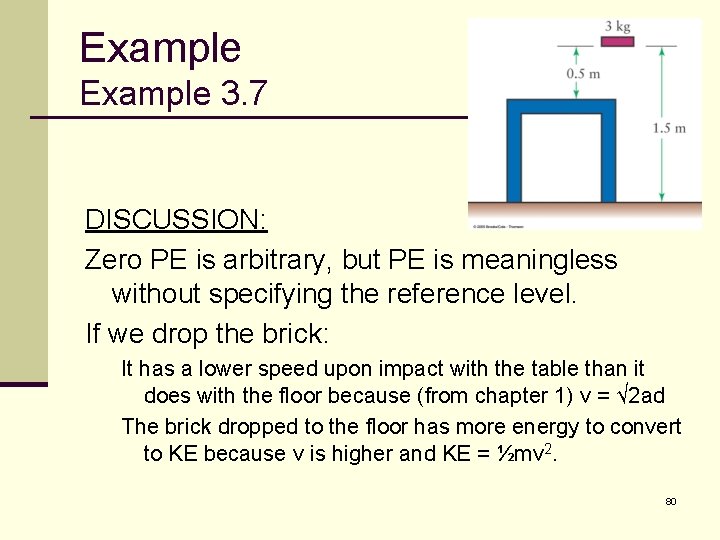
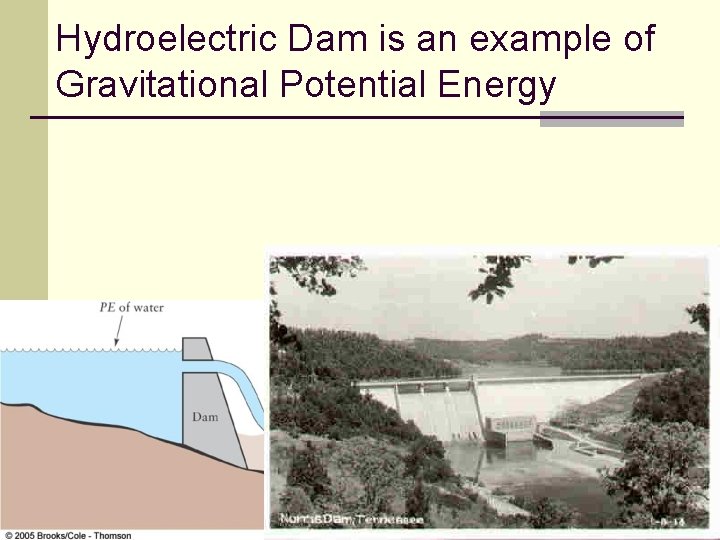
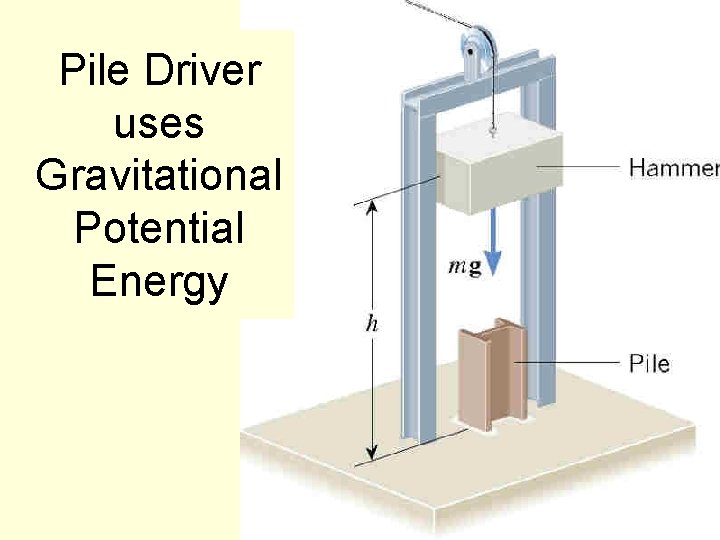
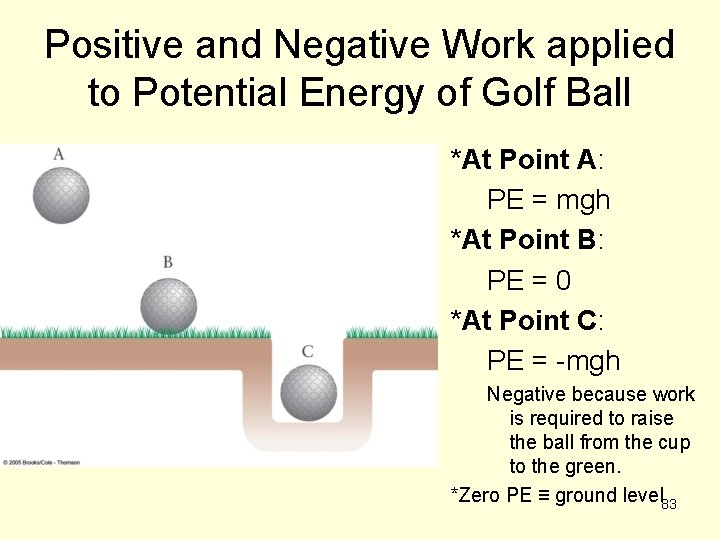
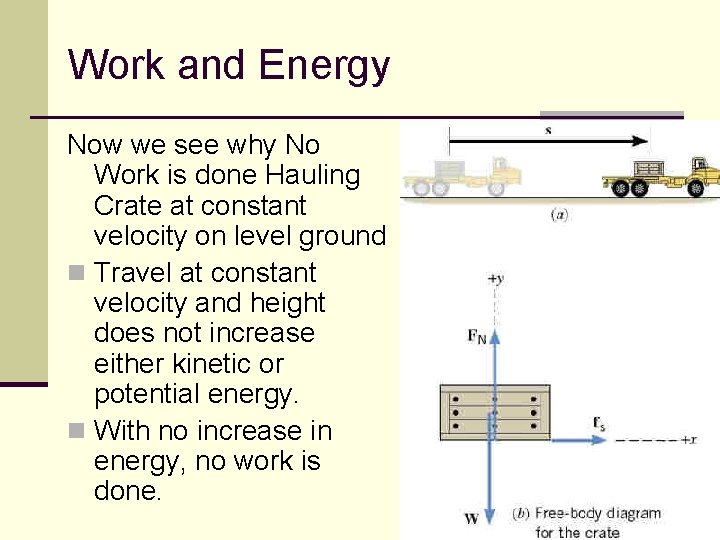
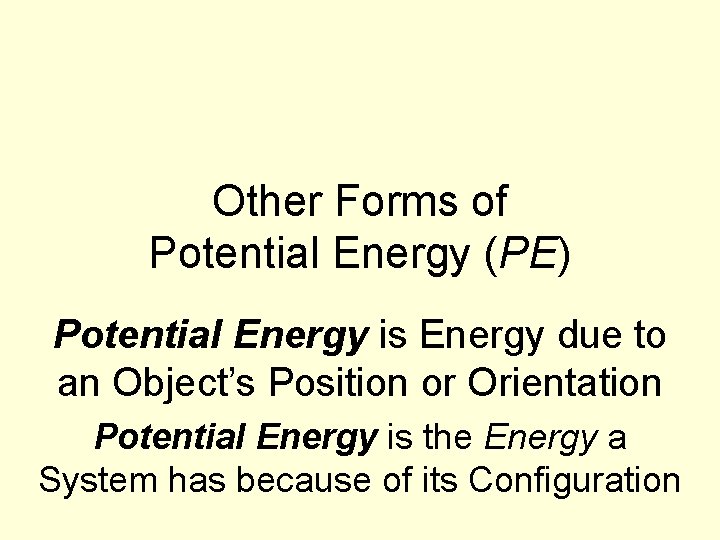
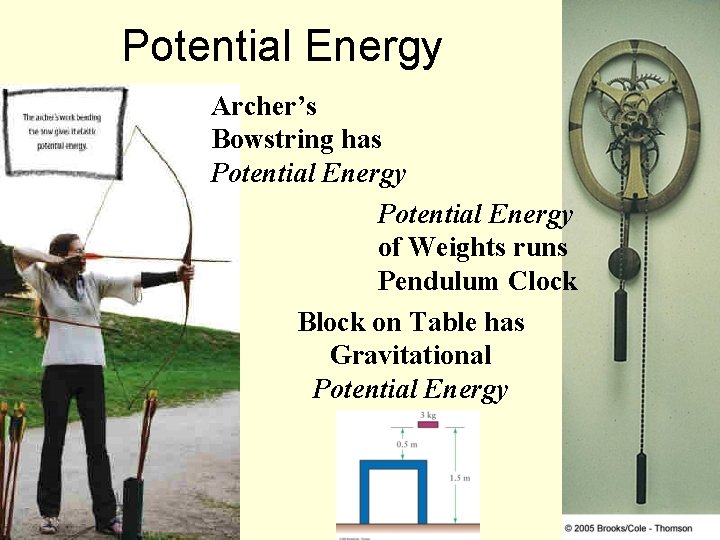
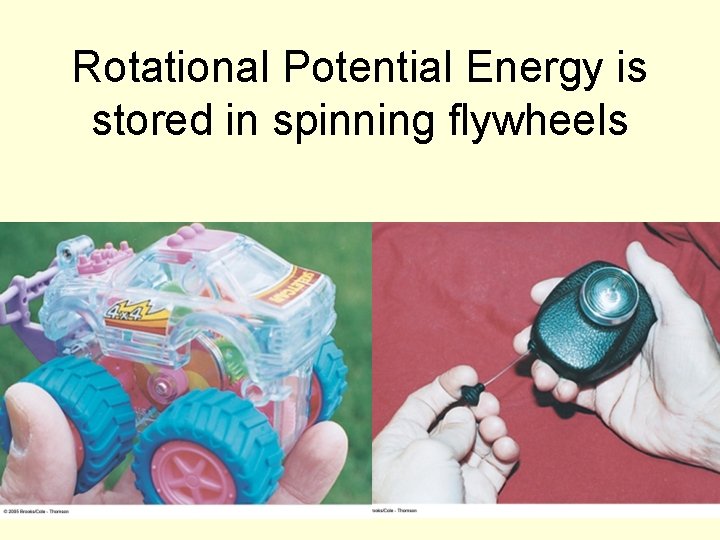
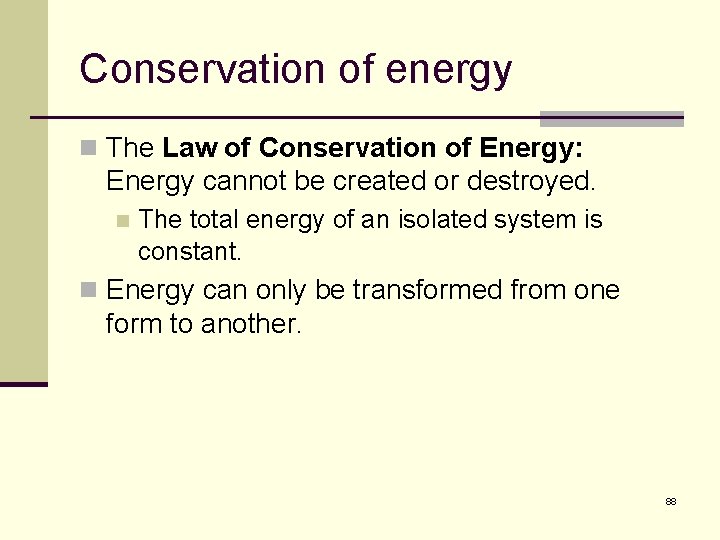
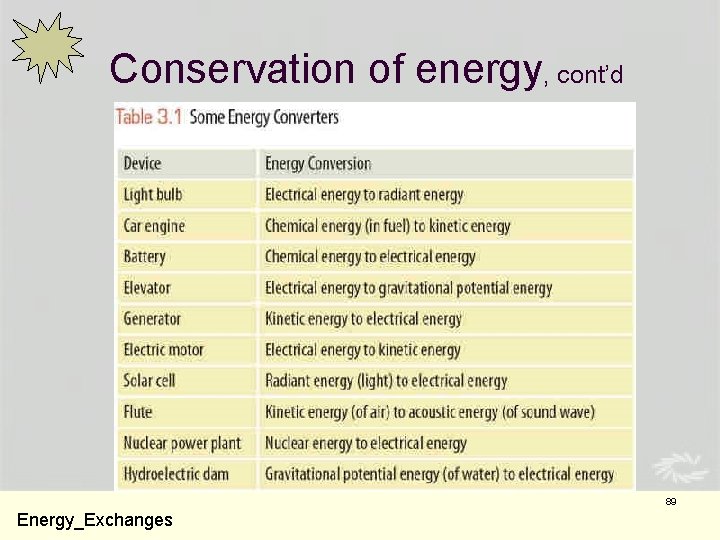
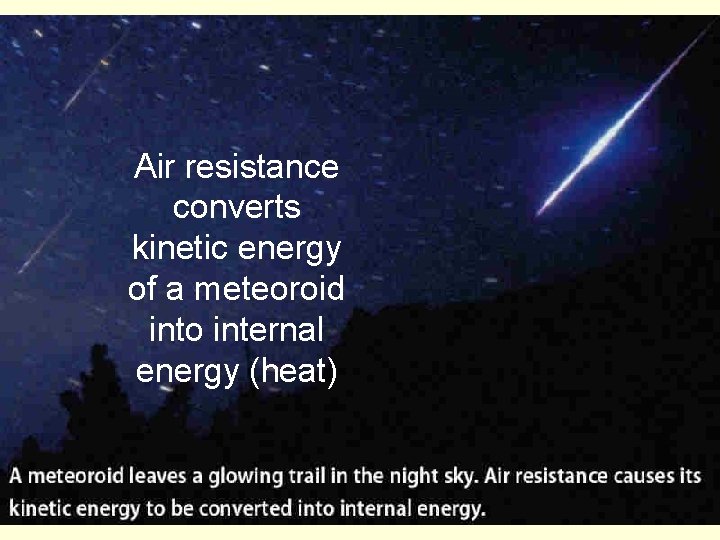
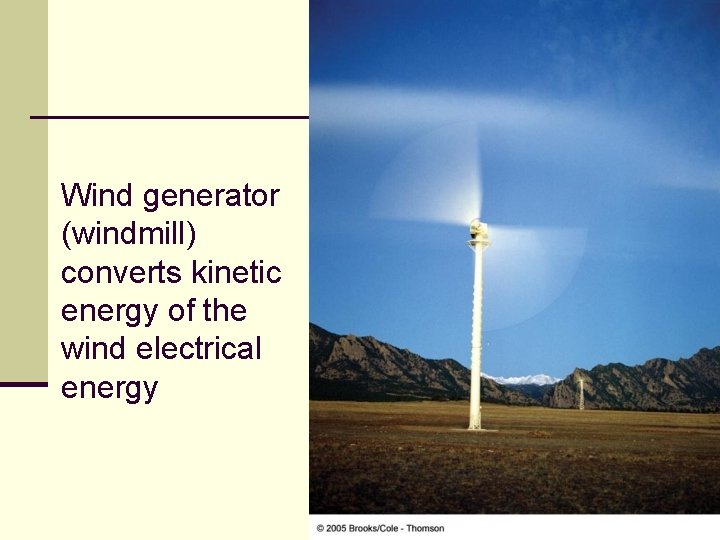
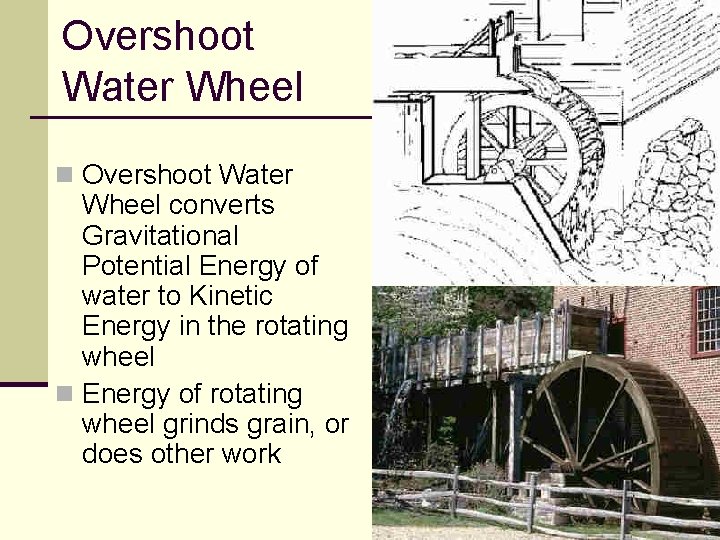
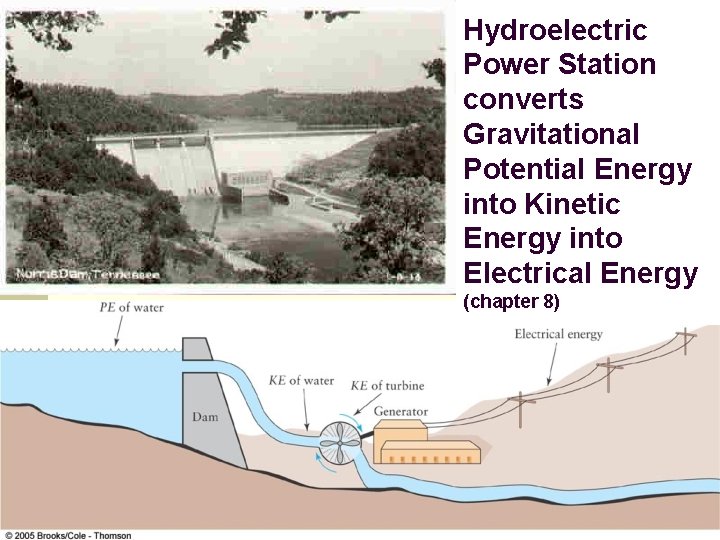
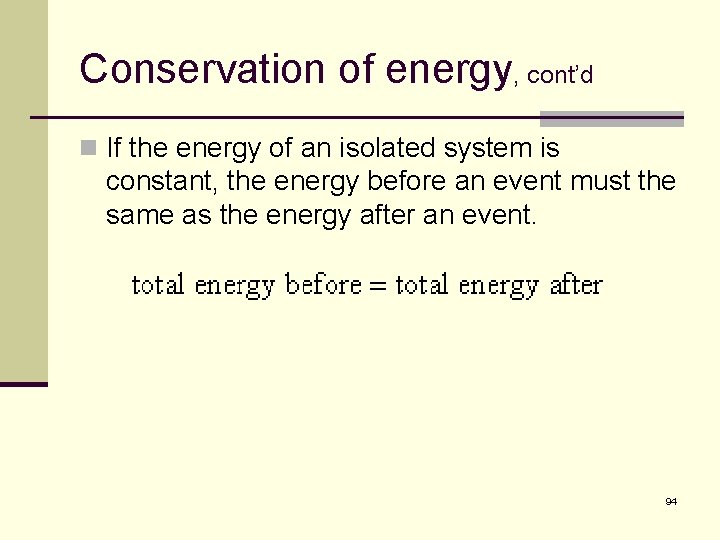
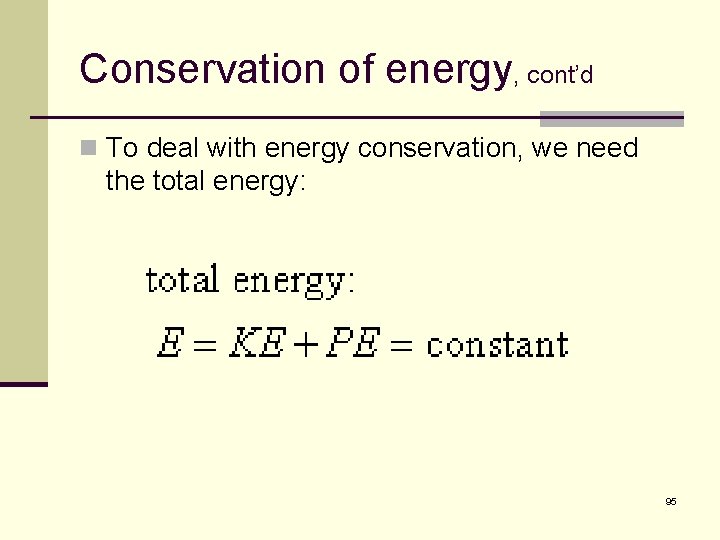
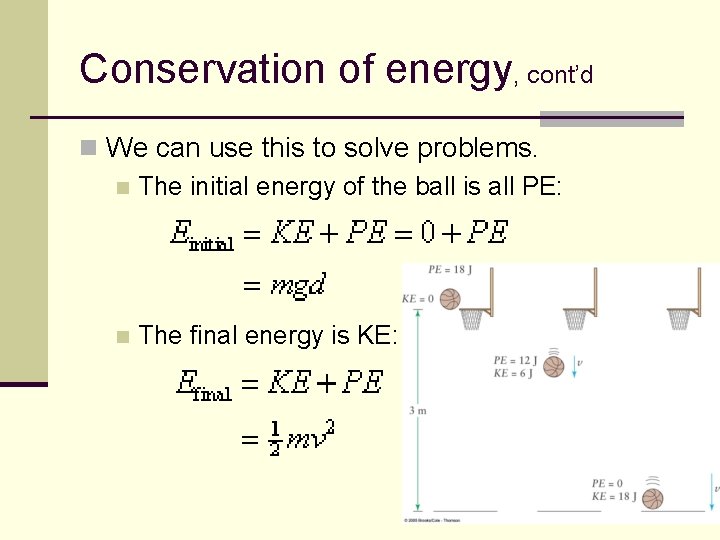
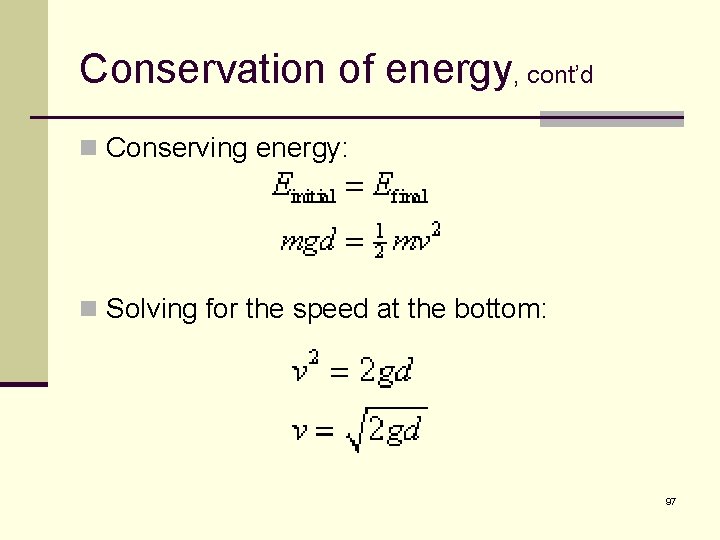
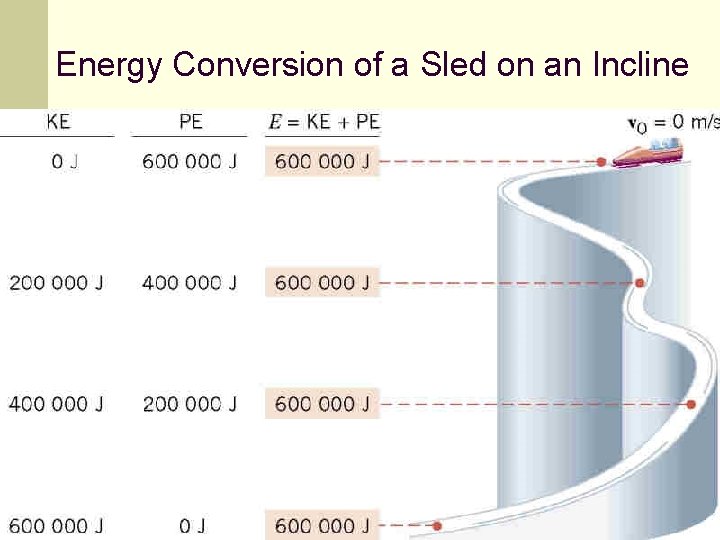
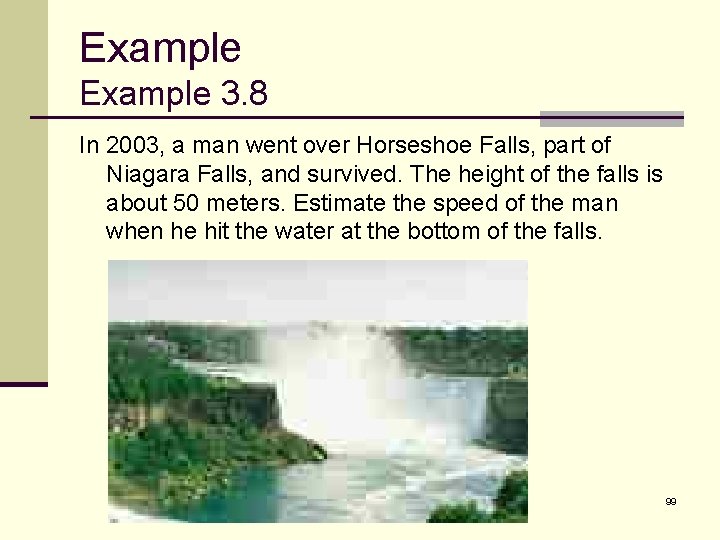
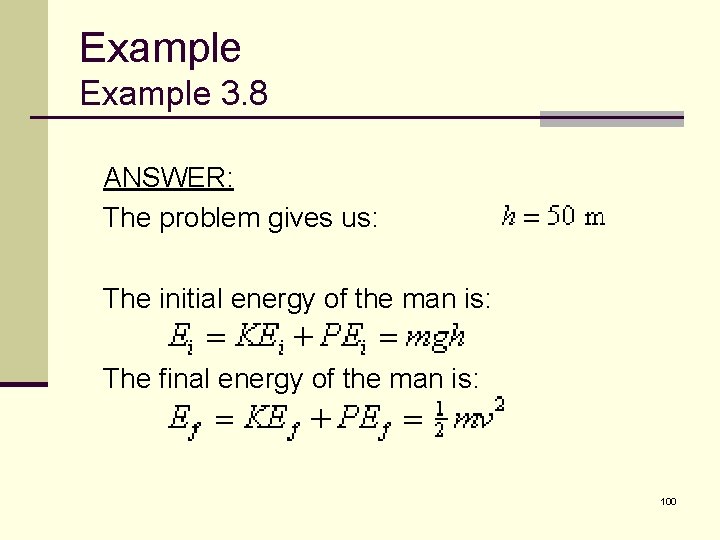
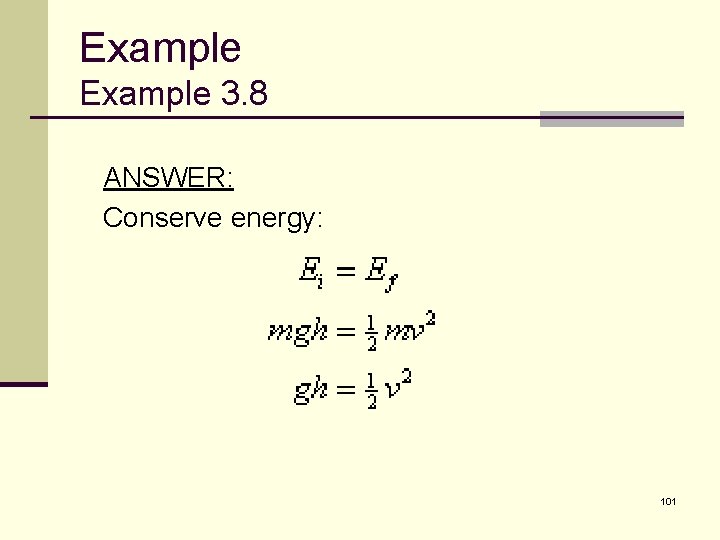
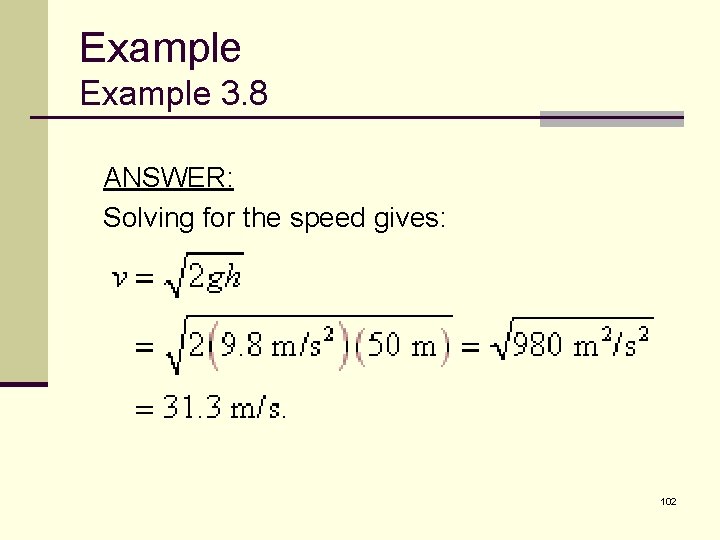
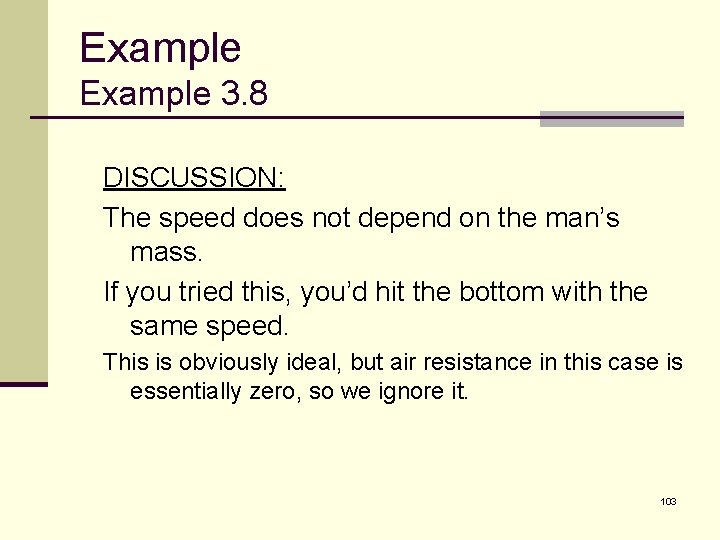
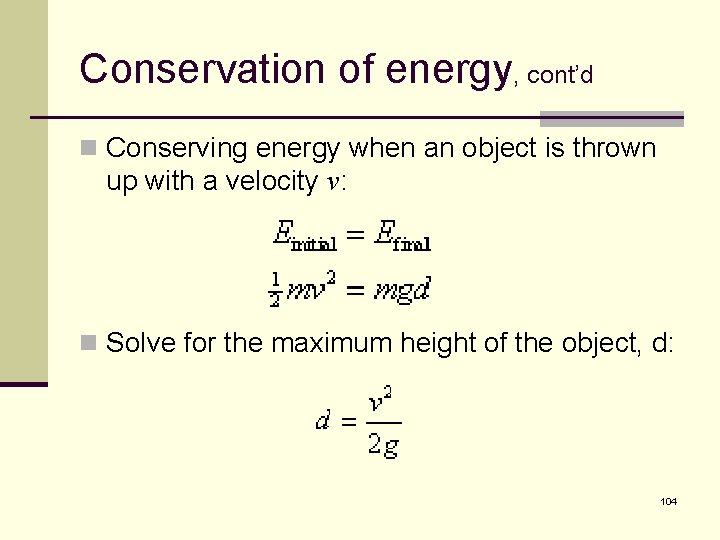
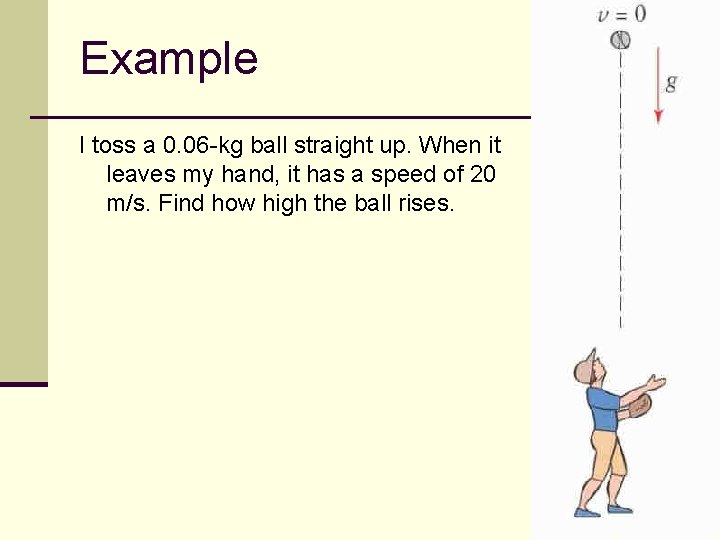
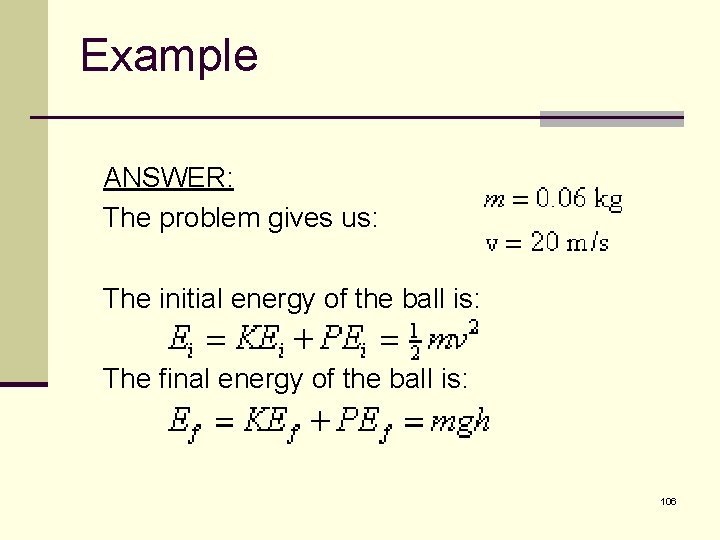
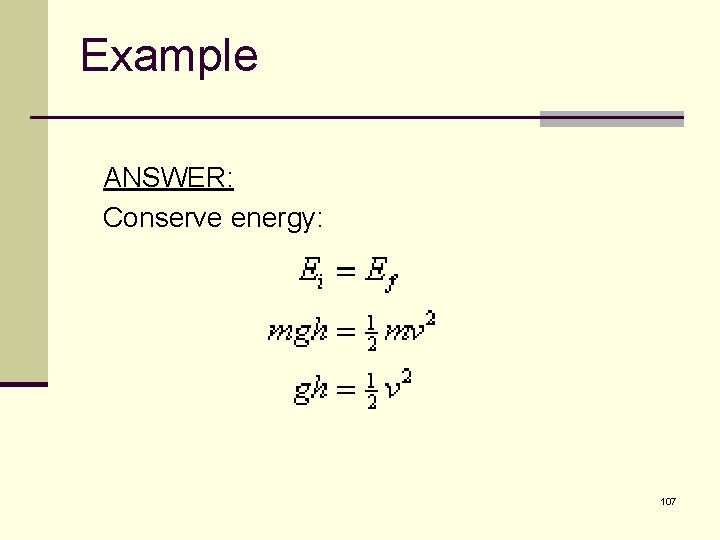
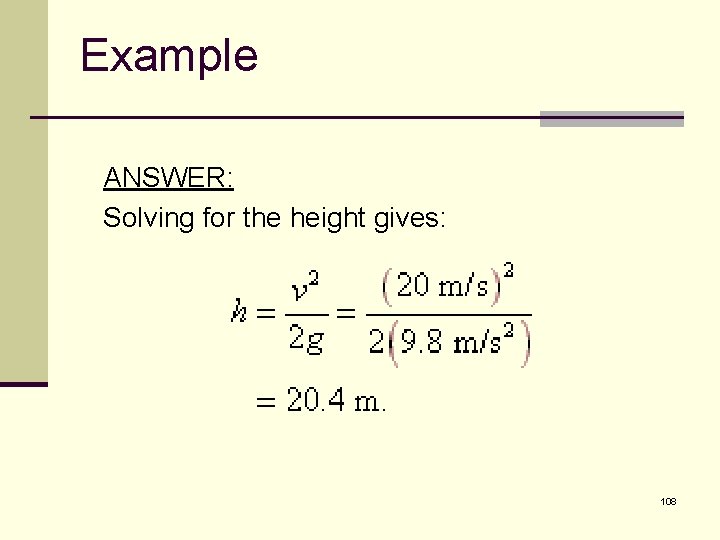
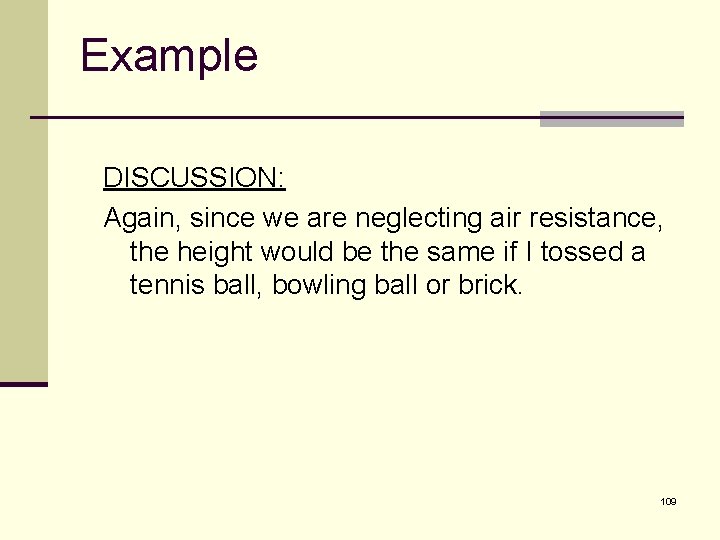
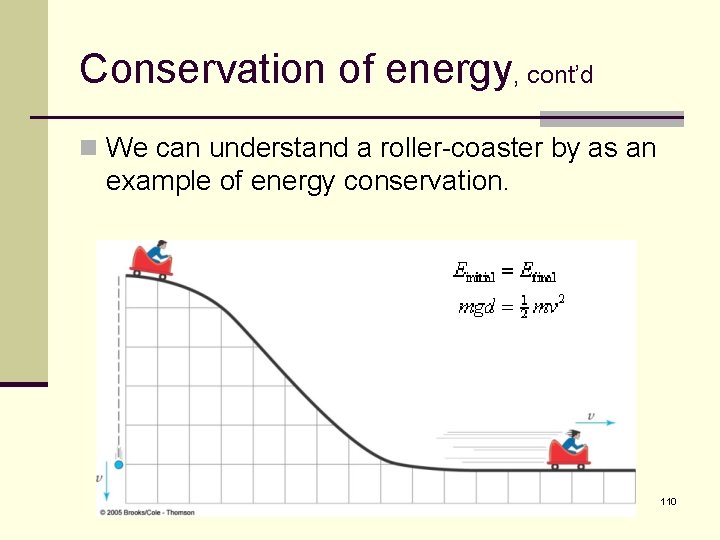
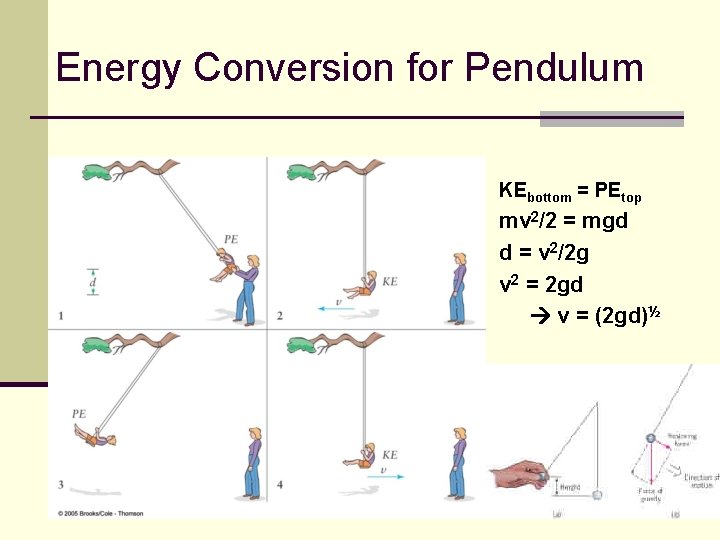
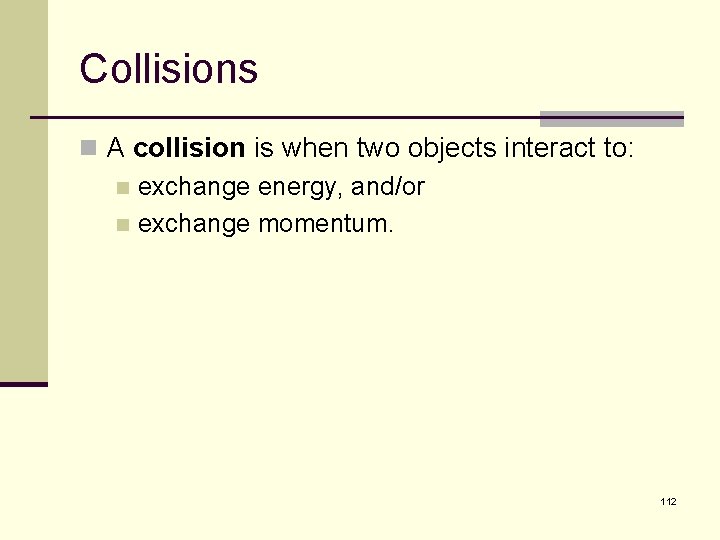
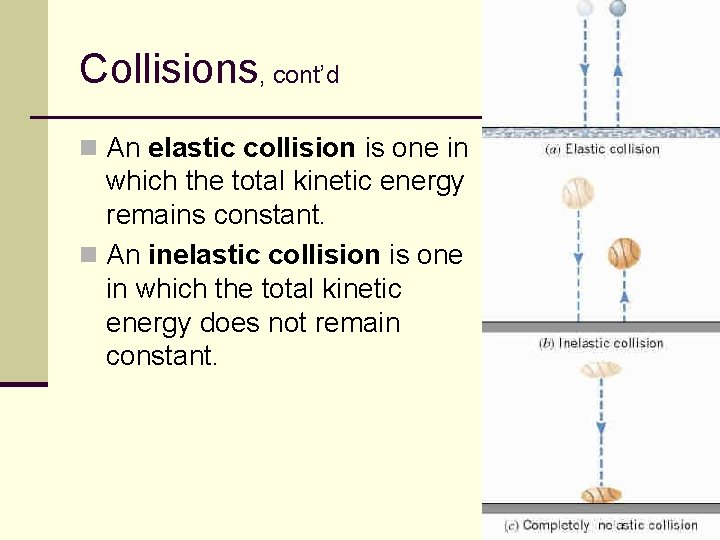
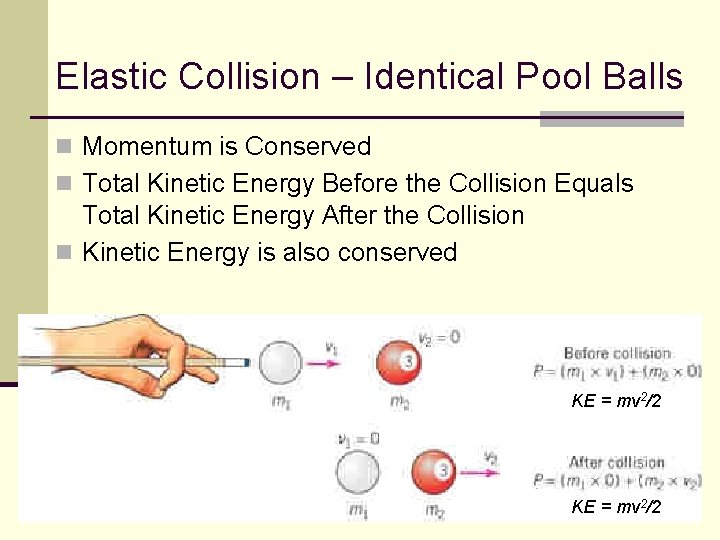
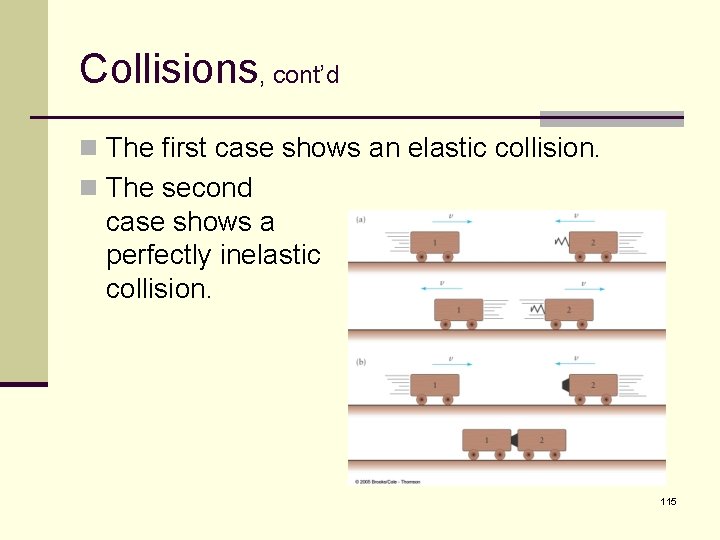
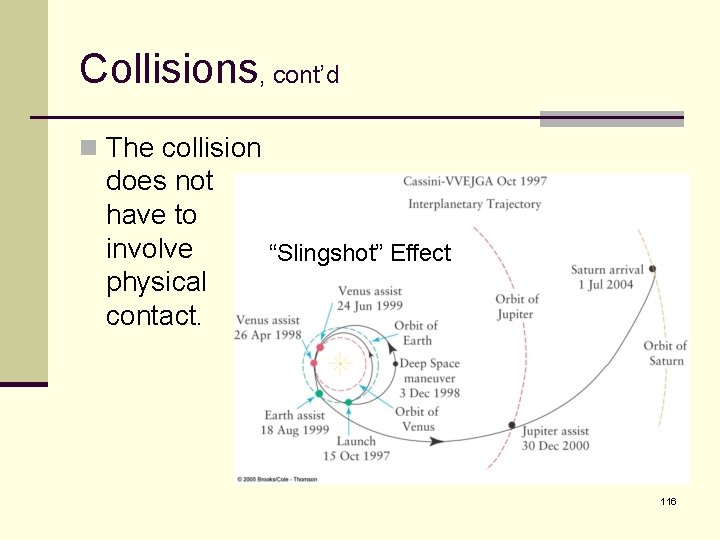
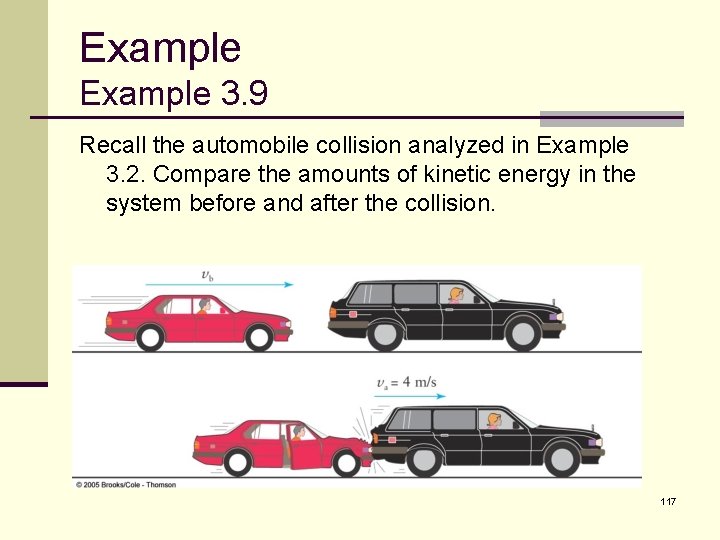
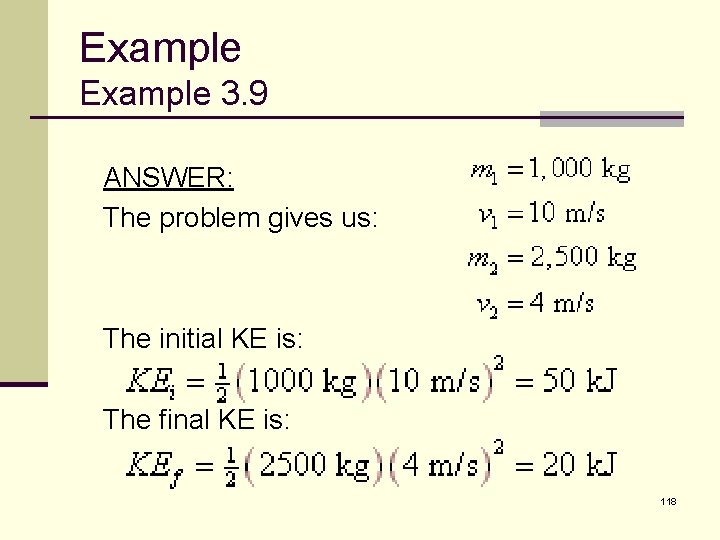
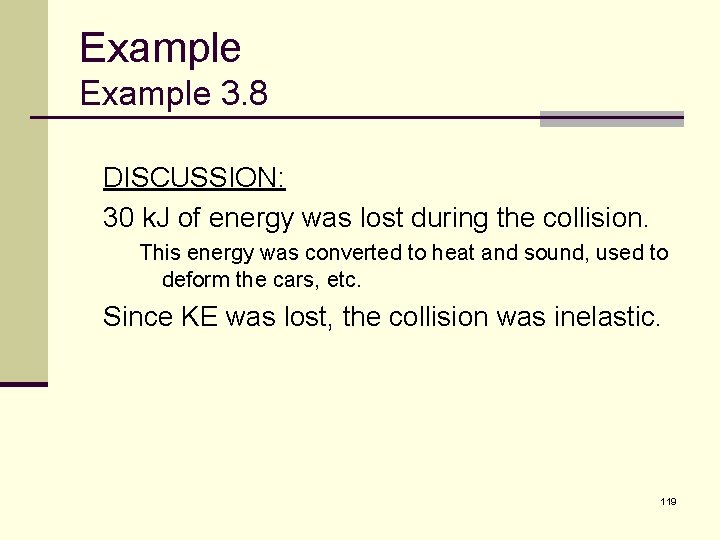
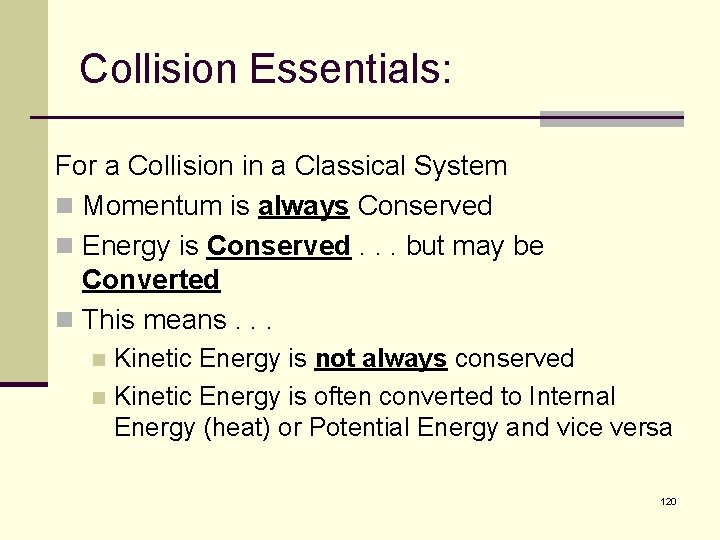
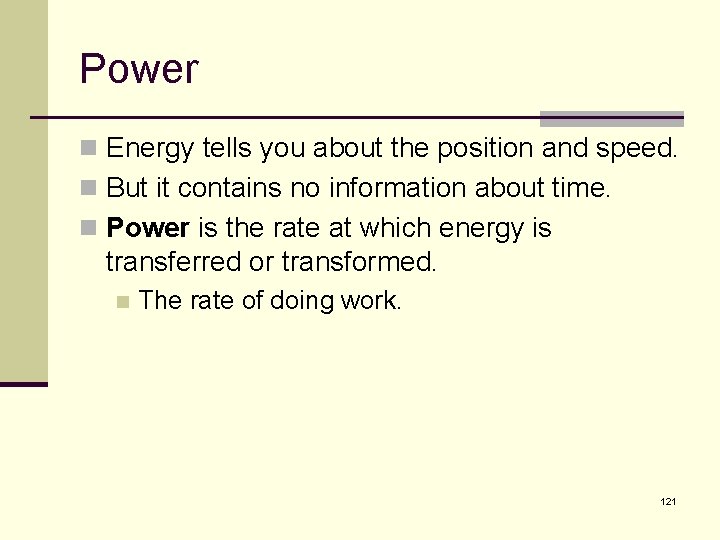
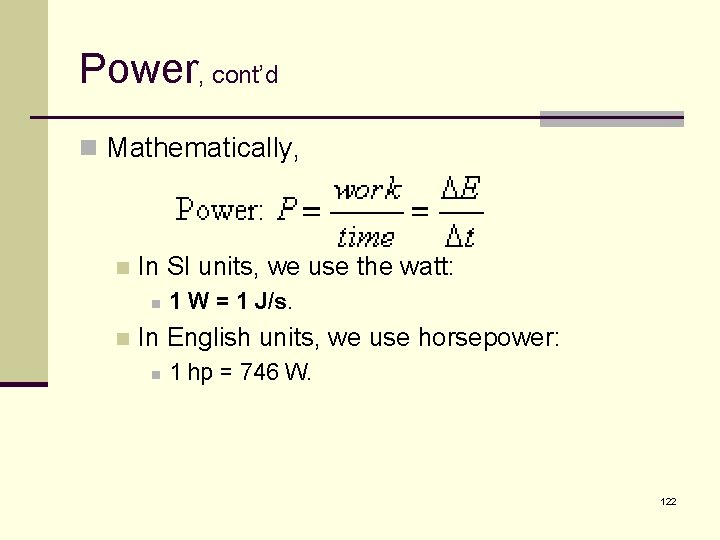
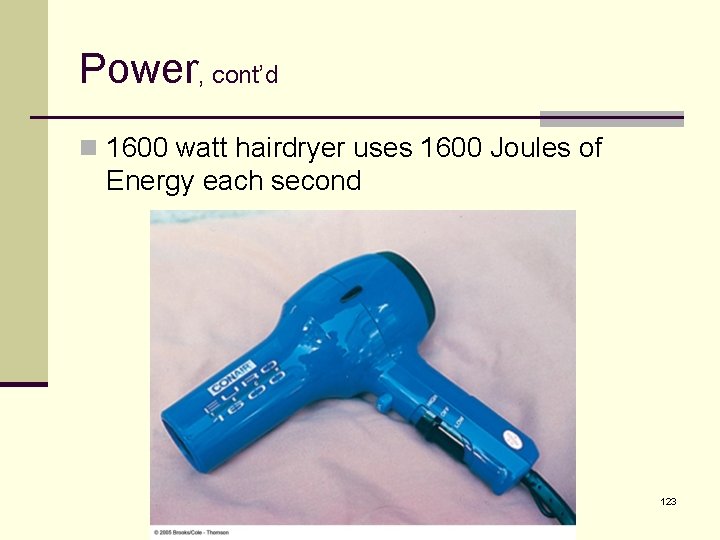
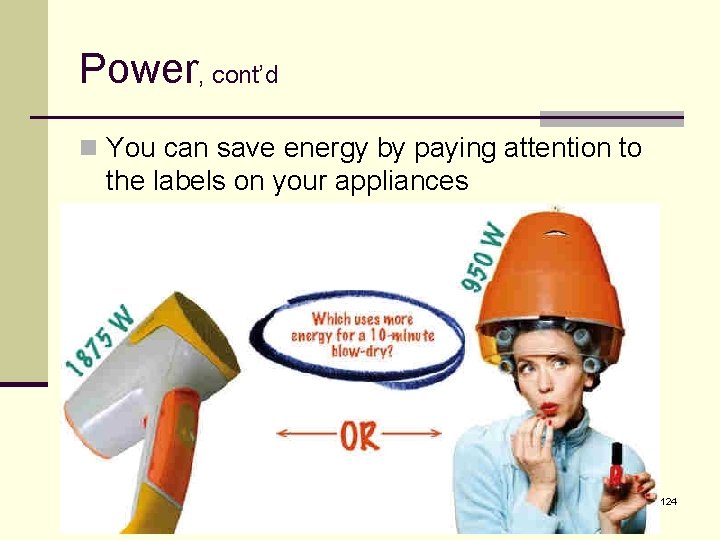
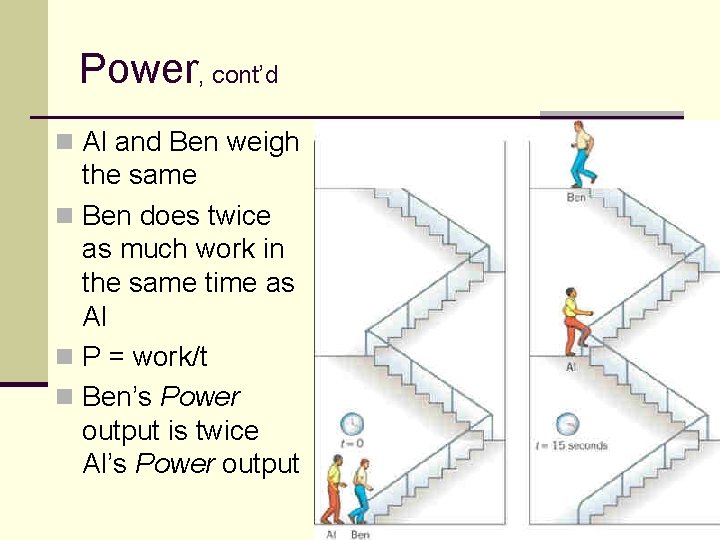
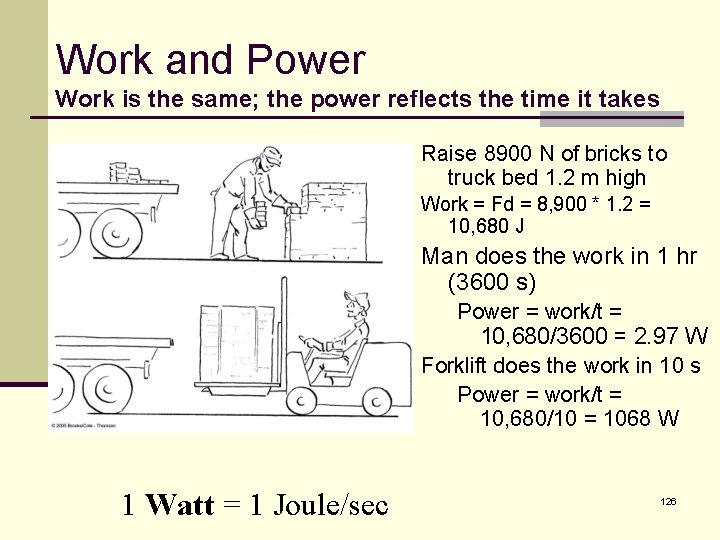
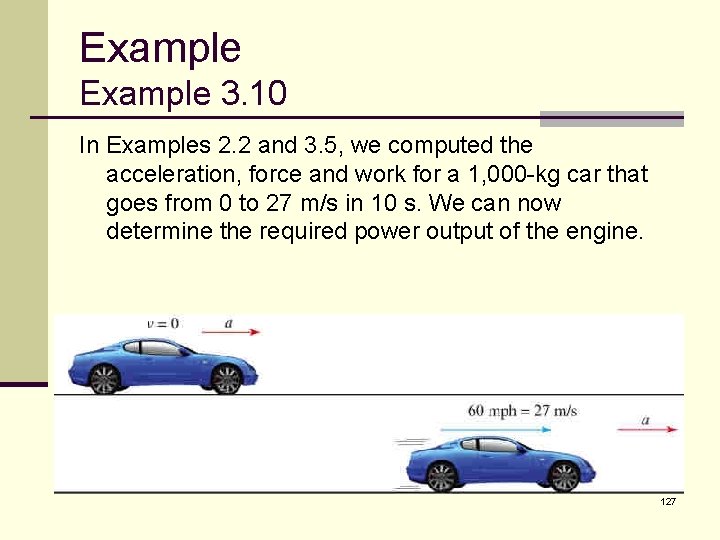
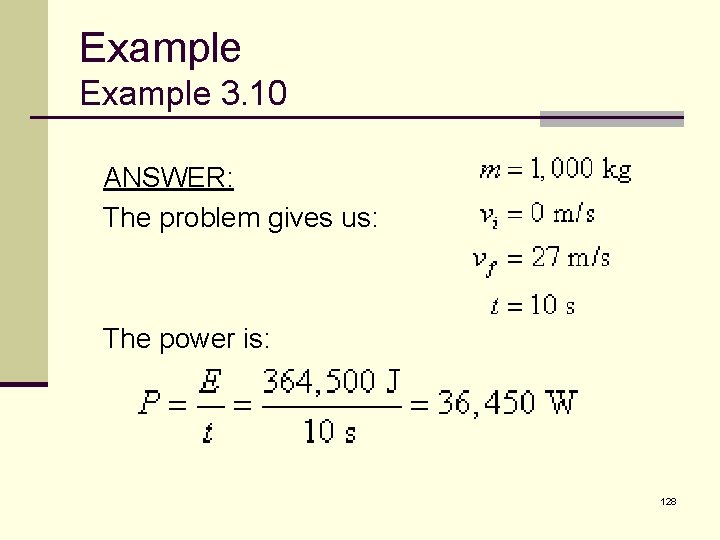
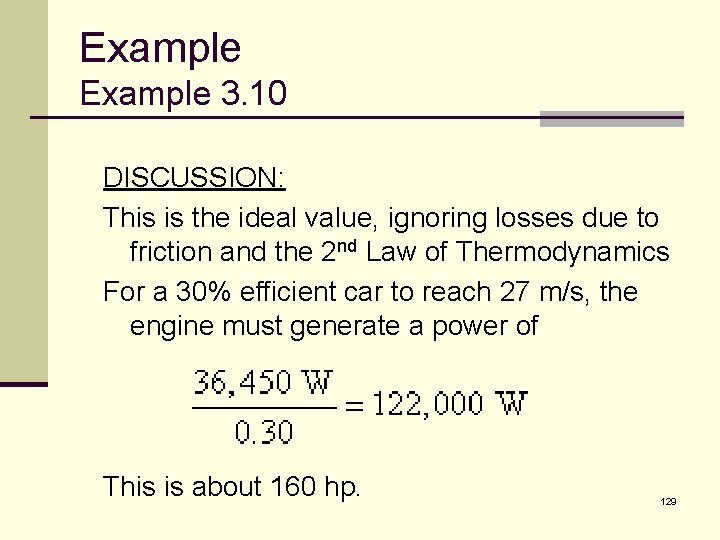
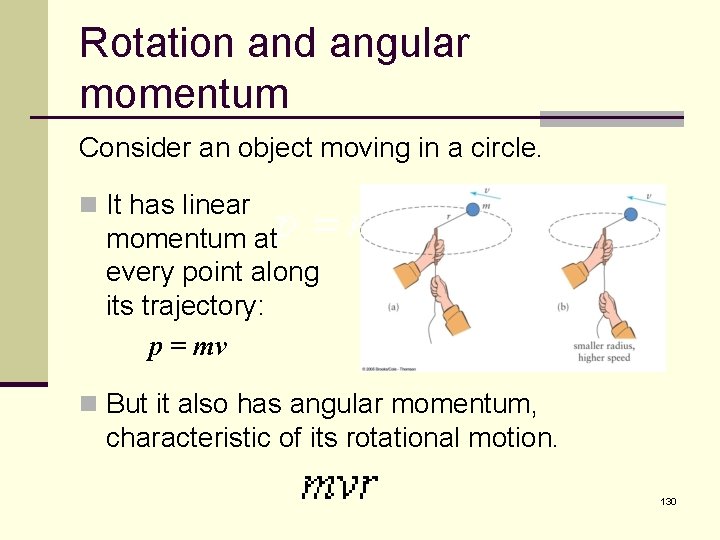
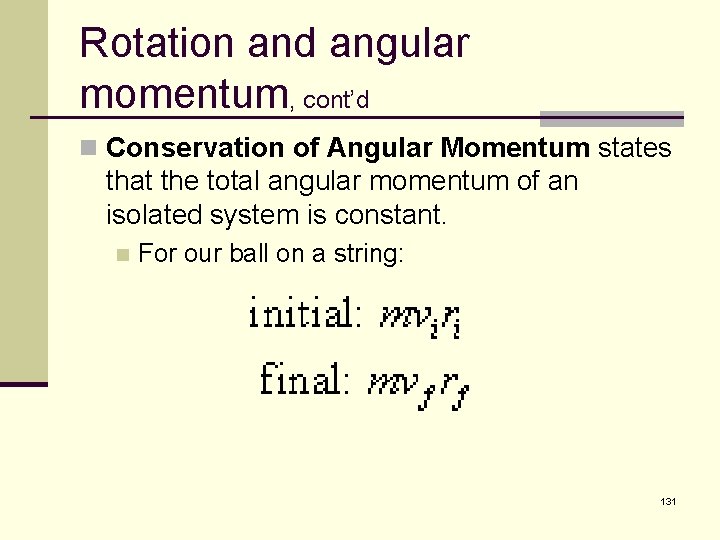
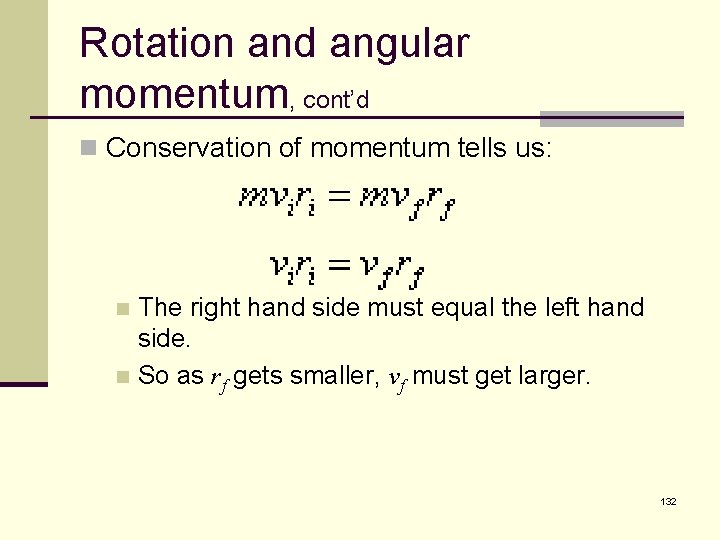
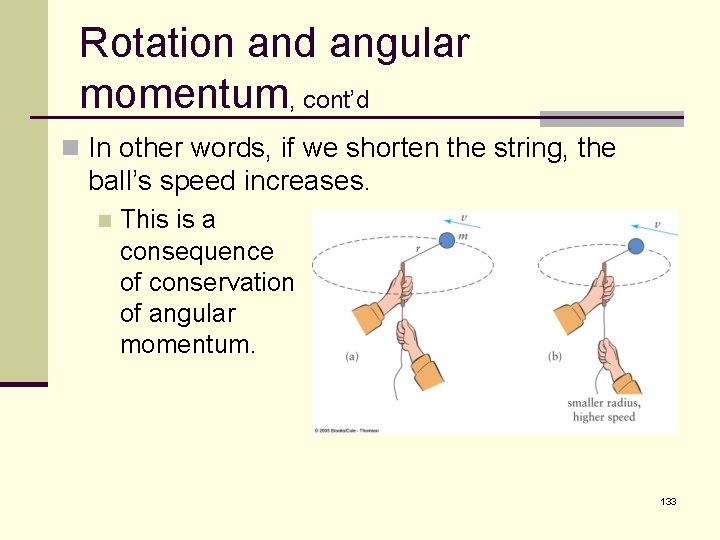
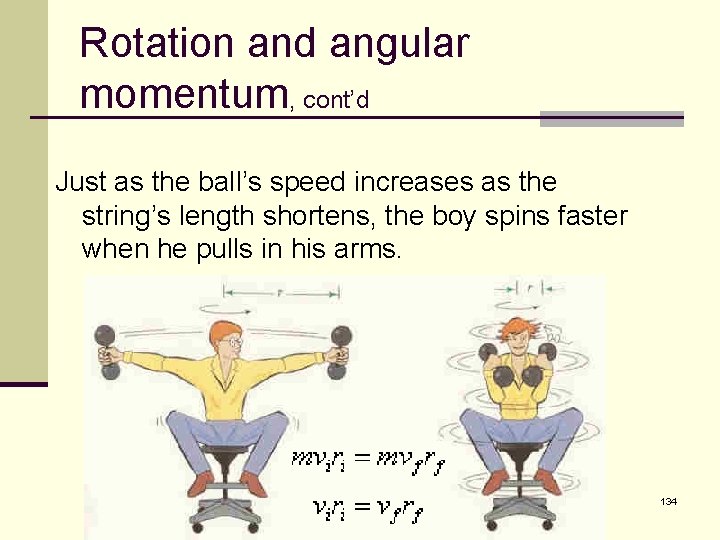
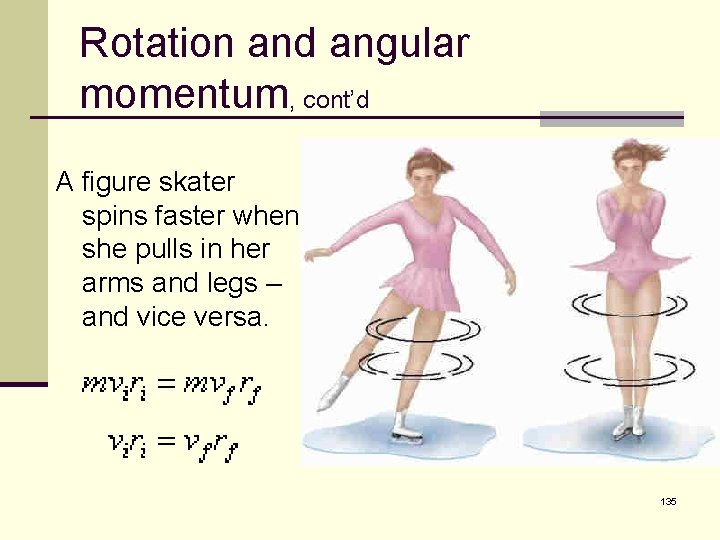
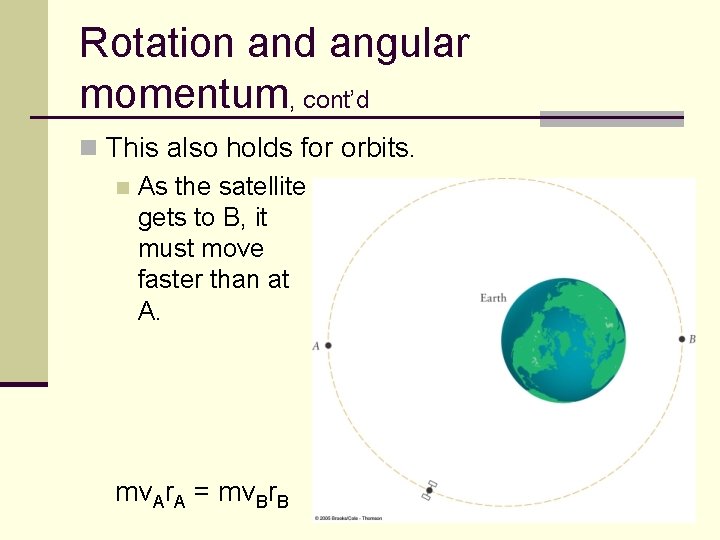
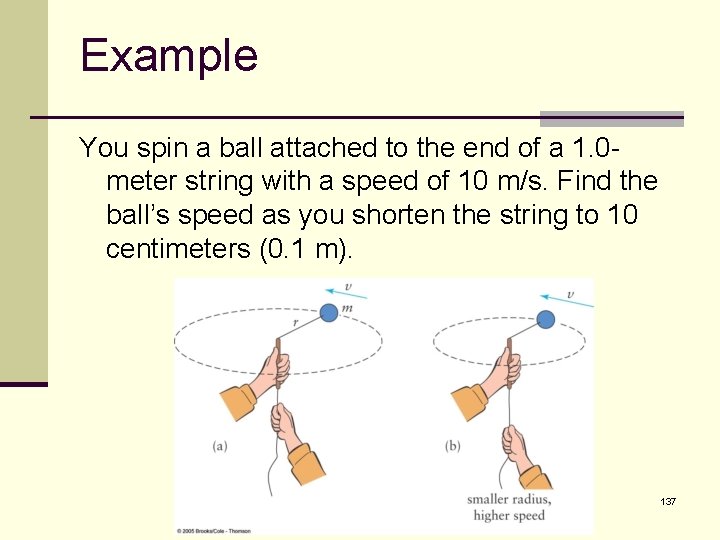
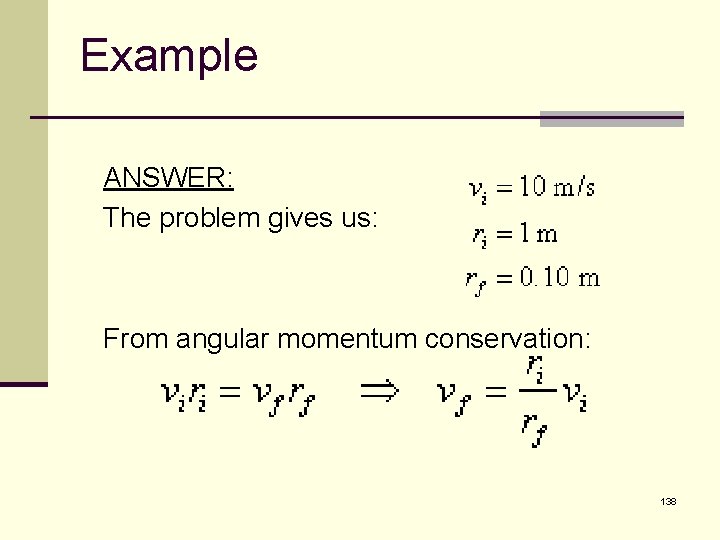
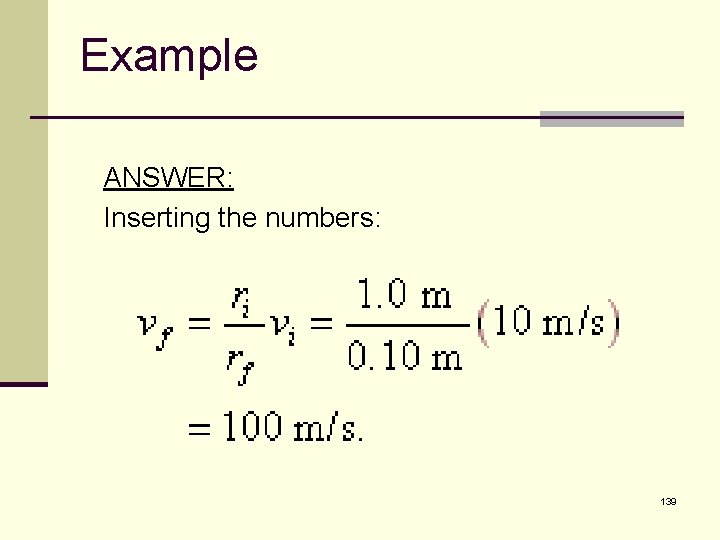
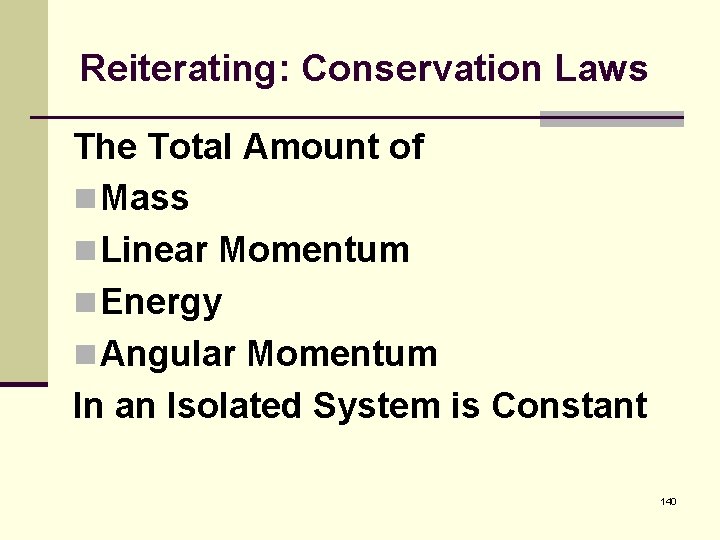
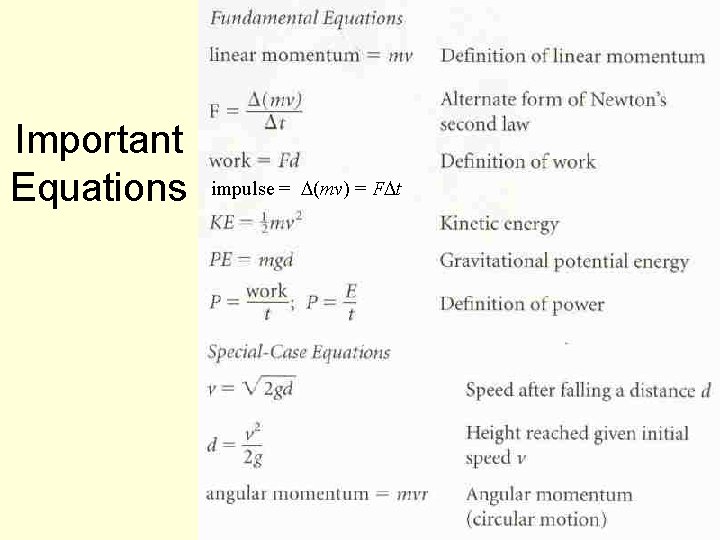
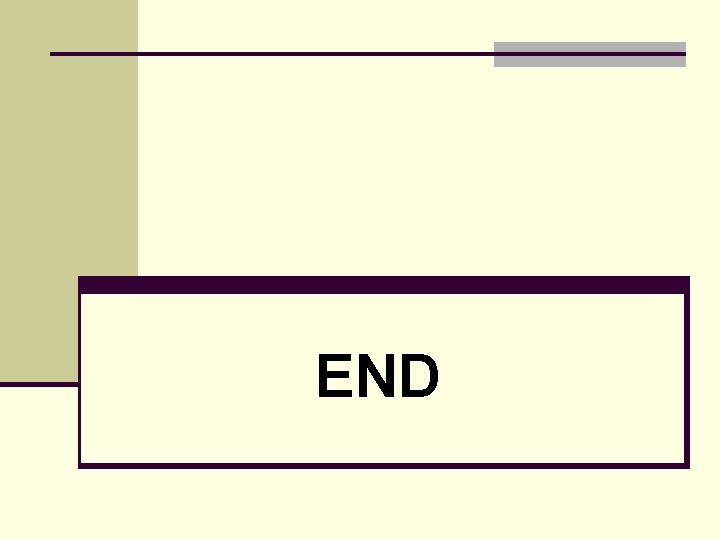
- Slides: 142
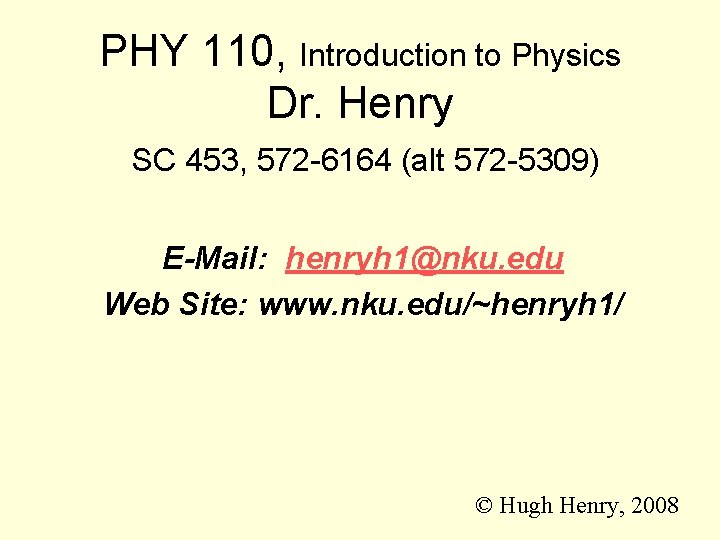
PHY 110, Introduction to Physics Dr. Henry SC 453, 572 -6164 (alt 572 -5309) E-Mail: henryh 1@nku. edu Web Site: www. nku. edu/~henryh 1/ © Hugh Henry, 2008
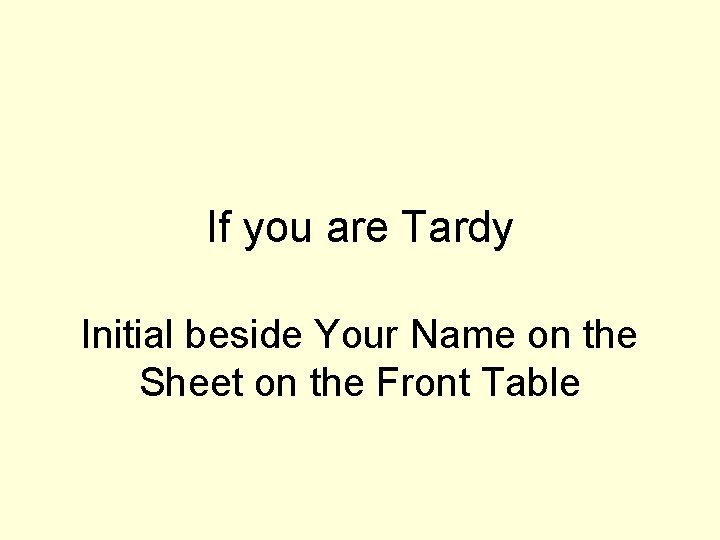
If you are Tardy Initial beside Your Name on the Sheet on the Front Table
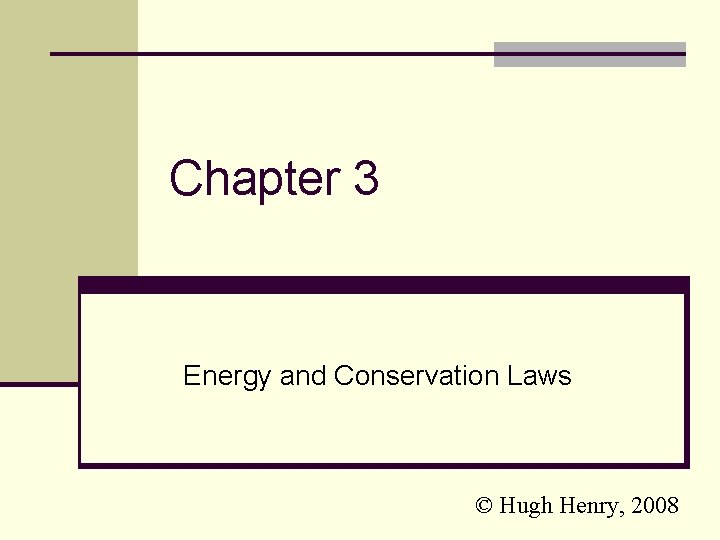
Chapter 3 Energy and Conservation Laws © Hugh Henry, 2008
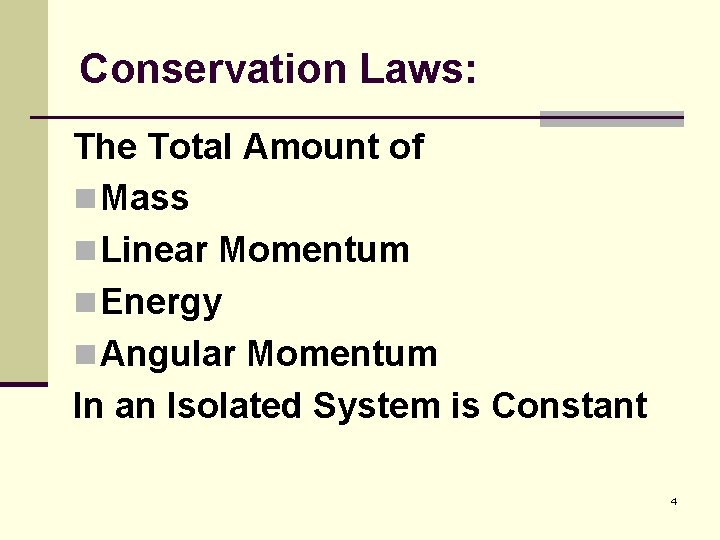
Conservation Laws: The Total Amount of n Mass n Linear Momentum n Energy n Angular Momentum In an Isolated System is Constant 4
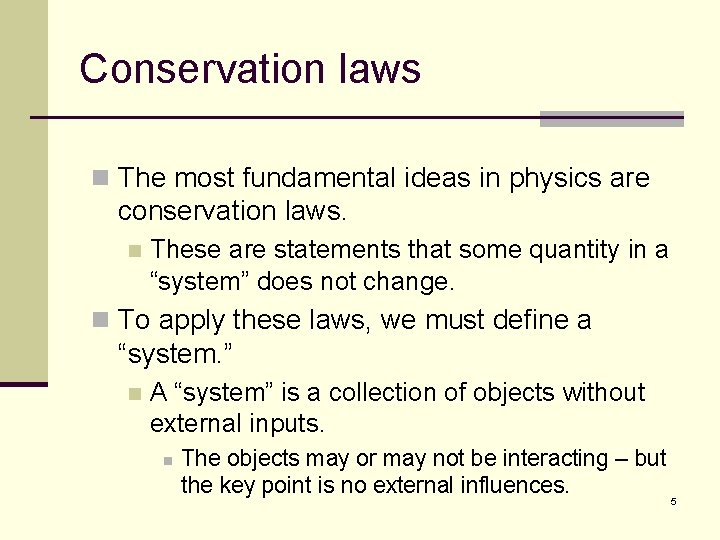
Conservation laws n The most fundamental ideas in physics are conservation laws. n These are statements that some quantity in a “system” does not change. n To apply these laws, we must define a “system. ” n A “system” is a collection of objects without external inputs. n The objects may or may not be interacting – but the key point is no external influences. 5
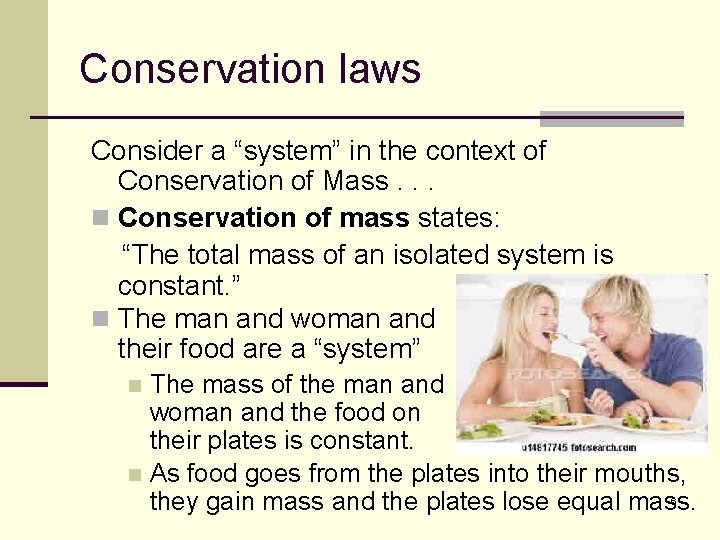
Conservation laws Consider a “system” in the context of Conservation of Mass. . . n Conservation of mass states: “The total mass of an isolated system is constant. ” n The man and woman and their food are a “system” The mass of the man and woman and the food on their plates is constant. n As food goes from the plates into their mouths, they gain mass and the plates lose equal mass. n 6
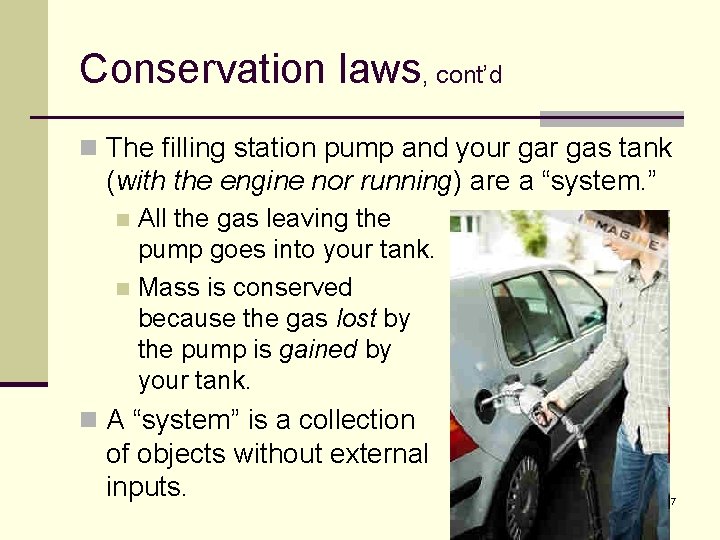
Conservation laws, cont’d n The filling station pump and your gas tank (with the engine nor running) are a “system. ” All the gas leaving the pump goes into your tank. n Mass is conserved because the gas lost by the pump is gained by your tank. n n A “system” is a collection of objects without external inputs. 7
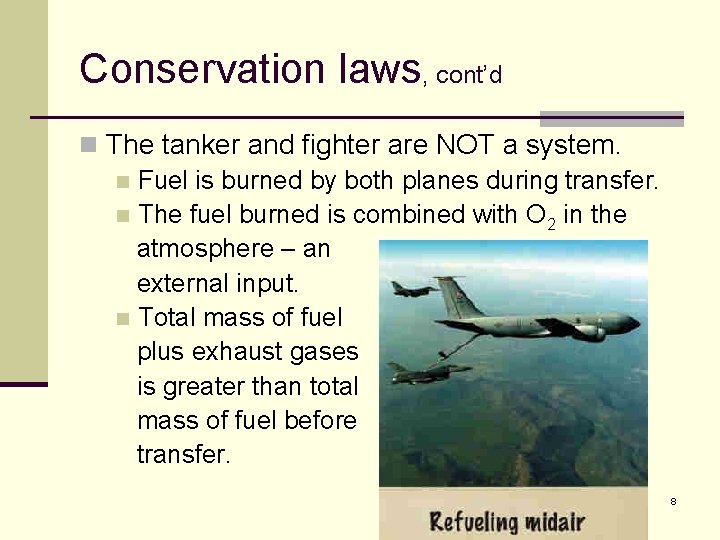
Conservation laws, cont’d n The tanker and fighter are NOT a system. n Fuel is burned by both planes during transfer. n The fuel burned is combined with O 2 in the atmosphere – an external input. n Total mass of fuel plus exhaust gases is greater than total mass of fuel before transfer. 8
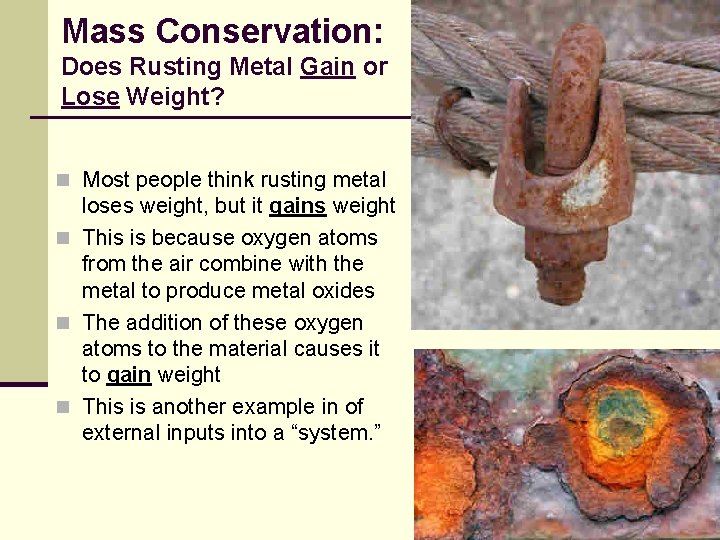
Mass Conservation: Does Rusting Metal Gain or Lose Weight? n Most people think rusting metal loses weight, but it gains weight n This is because oxygen atoms from the air combine with the metal to produce metal oxides n The addition of these oxygen atoms to the material causes it to gain weight n This is another example in of external inputs into a “system. ” 9
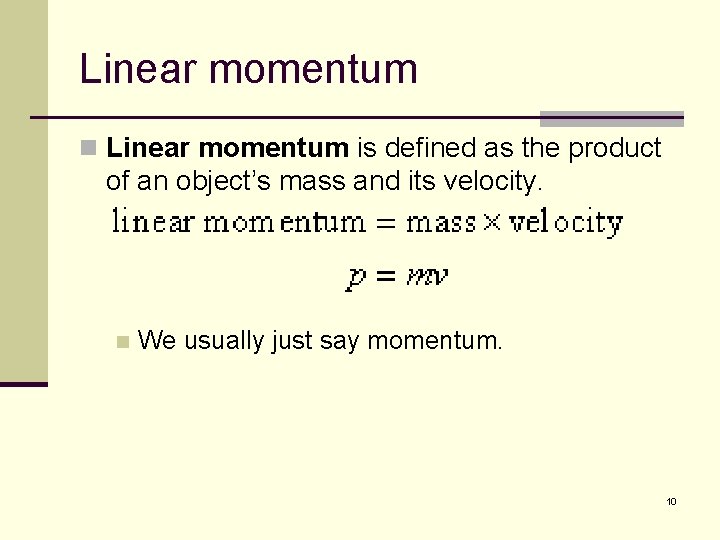
Linear momentum n Linear momentum is defined as the product of an object’s mass and its velocity. n We usually just say momentum. 10
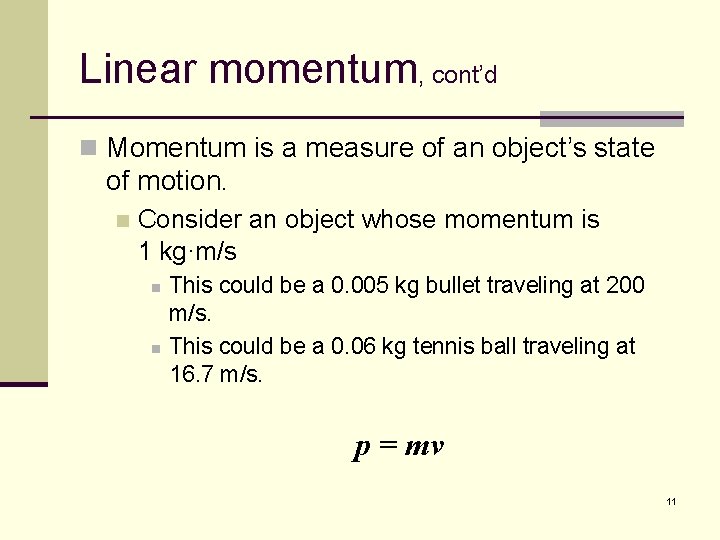
Linear momentum, cont’d n Momentum is a measure of an object’s state of motion. n Consider an object whose momentum is 1 kg·m/s n n This could be a 0. 005 kg bullet traveling at 200 m/s. This could be a 0. 06 kg tennis ball traveling at 16. 7 m/s. p = mv 11
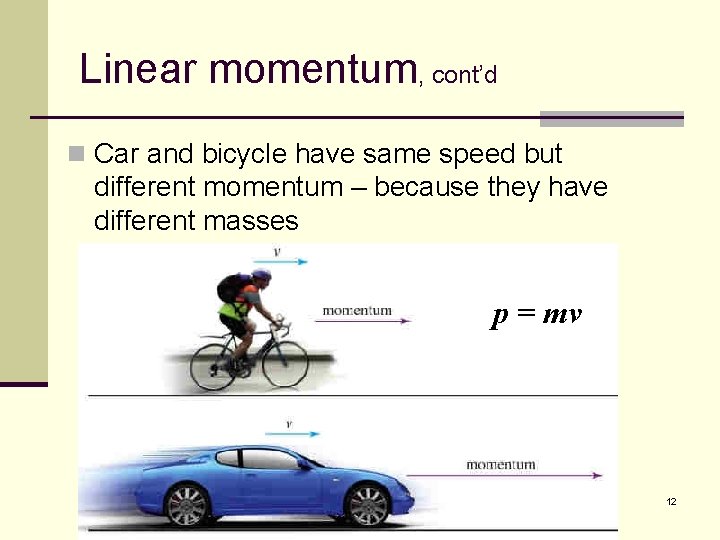
Linear momentum, cont’d n Car and bicycle have same speed but different momentum – because they have different masses p = mv 12
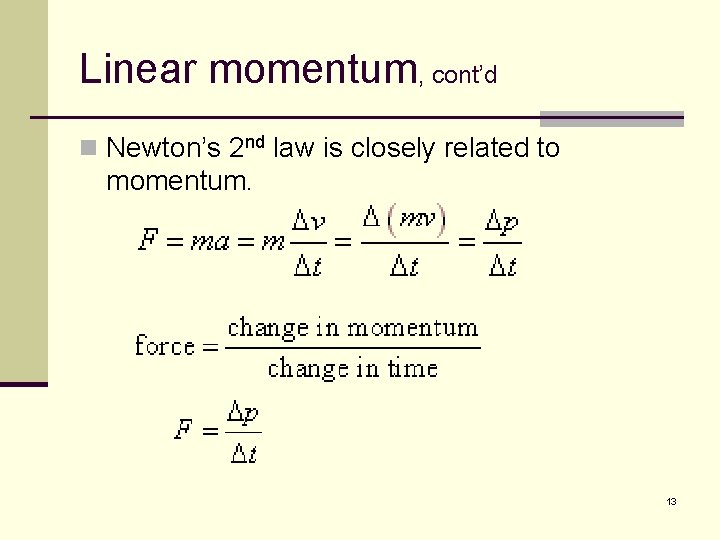
Linear momentum, cont’d n Newton’s 2 nd law is closely related to momentum. 13
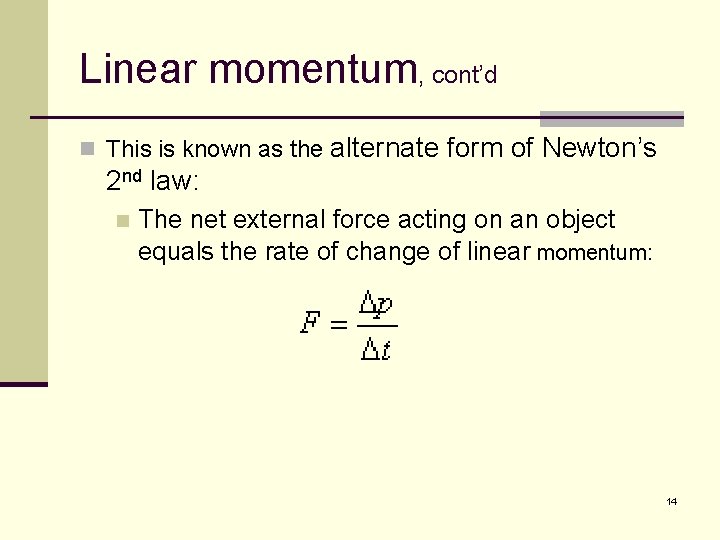
Linear momentum, cont’d n This is known as the alternate form of Newton’s 2 nd law: n The net external force acting on an object equals the rate of change of linear momentum: 14
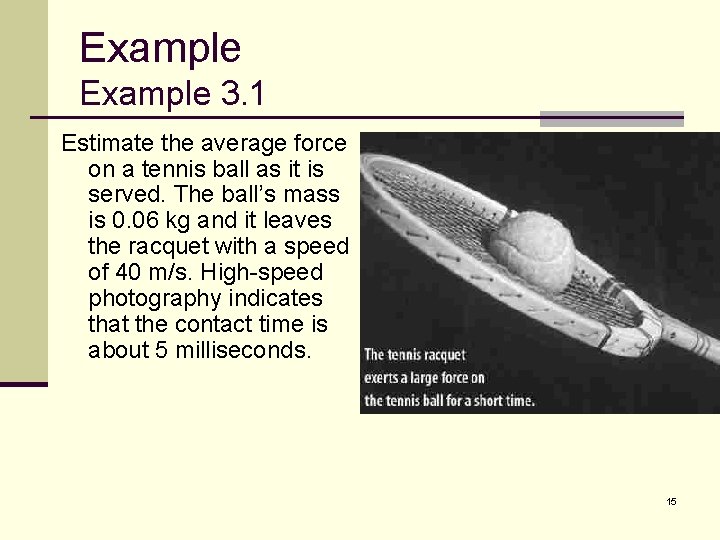
Example 3. 1 Estimate the average force on a tennis ball as it is served. The ball’s mass is 0. 06 kg and it leaves the racquet with a speed of 40 m/s. High-speed photography indicates that the contact time is about 5 milliseconds. 15
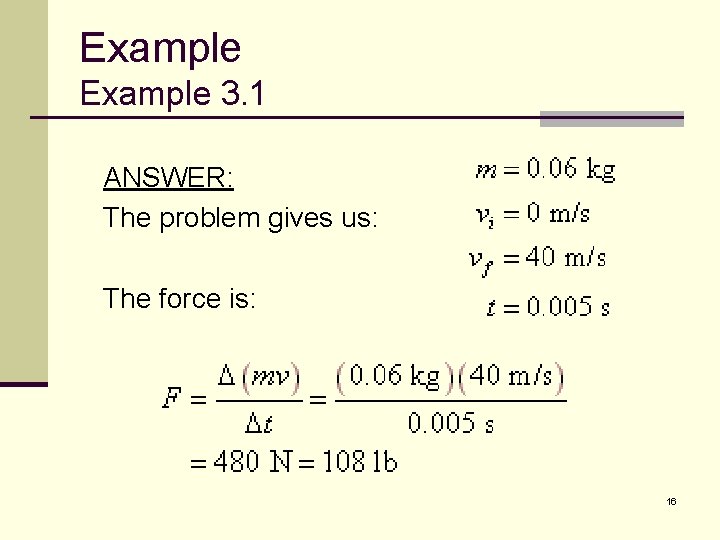
Example 3. 1 ANSWER: The problem gives us: The force is: 16
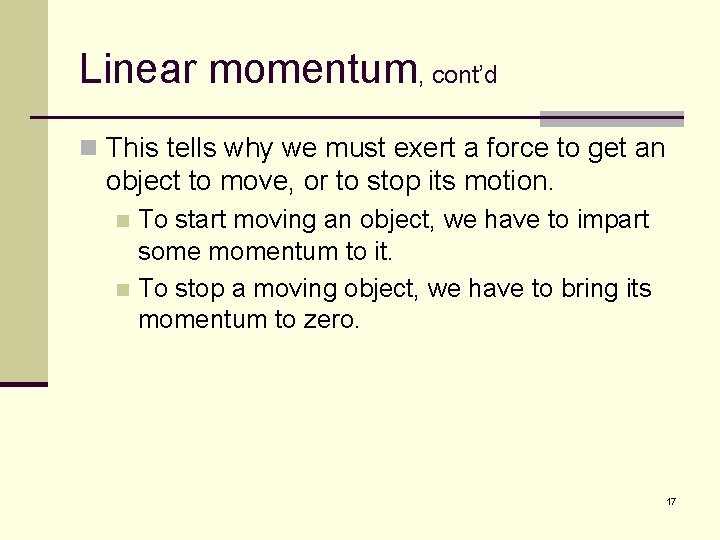
Linear momentum, cont’d n This tells why we must exert a force to get an object to move, or to stop its motion. To start moving an object, we have to impart some momentum to it. n To stop a moving object, we have to bring its momentum to zero. n 17
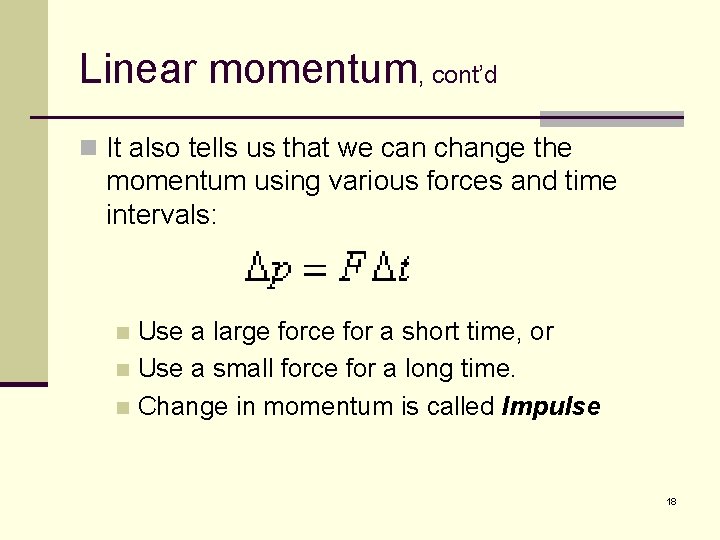
Linear momentum, cont’d n It also tells us that we can change the momentum using various forces and time intervals: Use a large force for a short time, or n Use a small force for a long time. n Change in momentum is called Impulse n 18
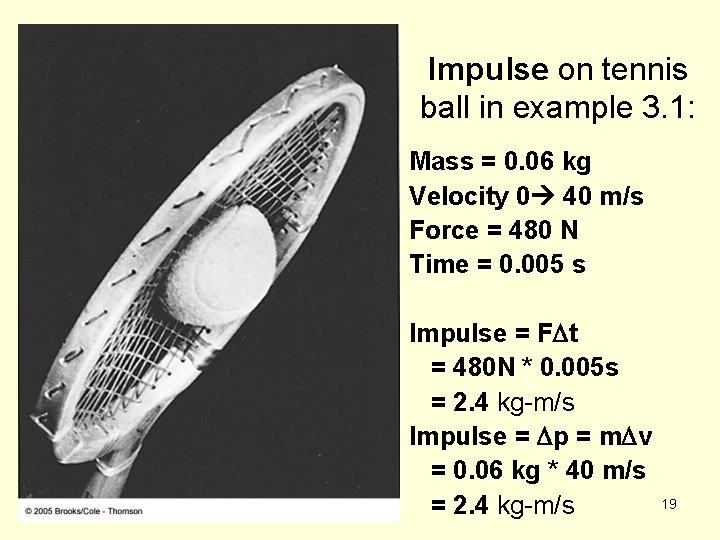
Impulse on tennis ball in example 3. 1: Mass = 0. 06 kg Velocity 0 40 m/s Force = 480 N Time = 0. 005 s Impulse = FDt = 480 N * 0. 005 s = 2. 4 kg-m/s Impulse = Dp = m. Dv = 0. 06 kg * 40 m/s = 2. 4 kg-m/s 19
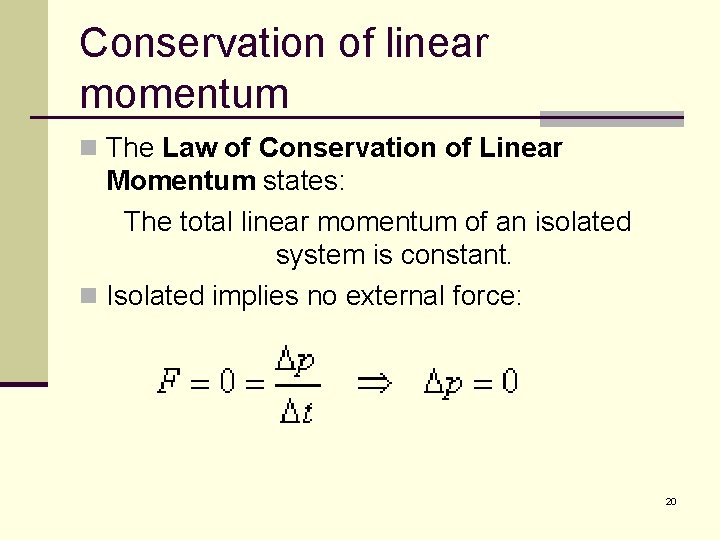
Conservation of linear momentum n The Law of Conservation of Linear Momentum states: The total linear momentum of an isolated system is constant. n Isolated implies no external force: 20
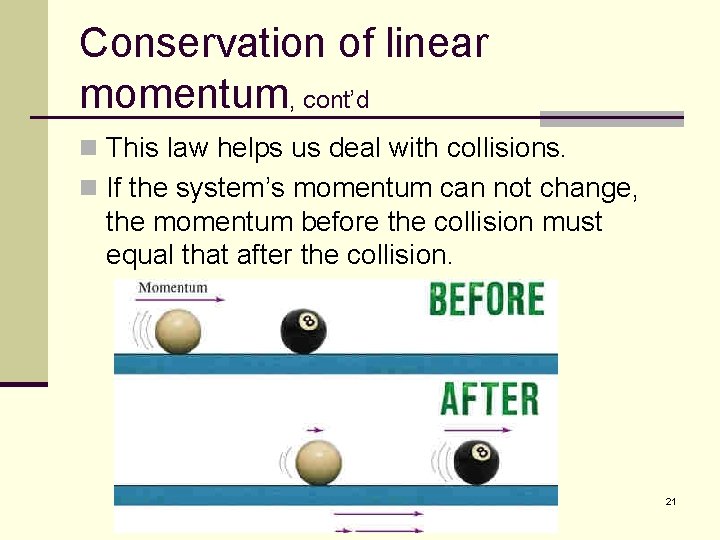
Conservation of linear momentum, cont’d n This law helps us deal with collisions. n If the system’s momentum can not change, the momentum before the collision must equal that after the collision. 21
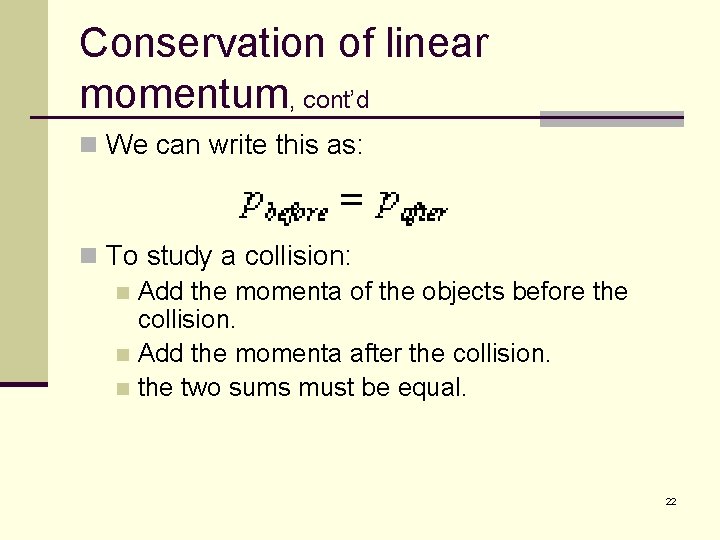
Conservation of linear momentum, cont’d n We can write this as: n To study a collision: n Add the momenta of the objects before the collision. n Add the momenta after the collision. n the two sums must be equal. 22
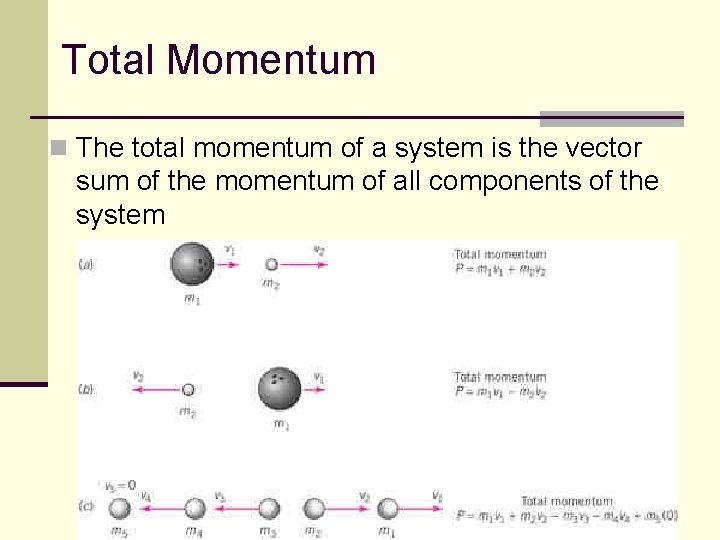
Total Momentum n The total momentum of a system is the vector sum of the momentum of all components of the system 23
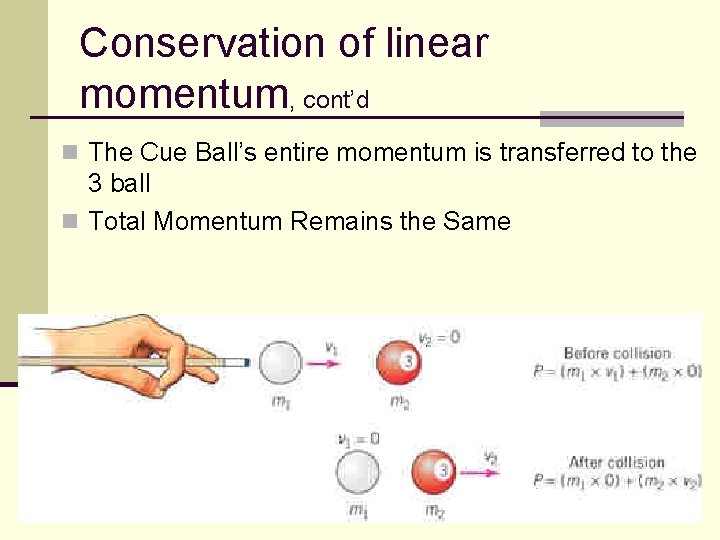
Conservation of linear momentum, cont’d n The Cue Ball’s entire momentum is transferred to the 3 ball n Total Momentum Remains the Same 24
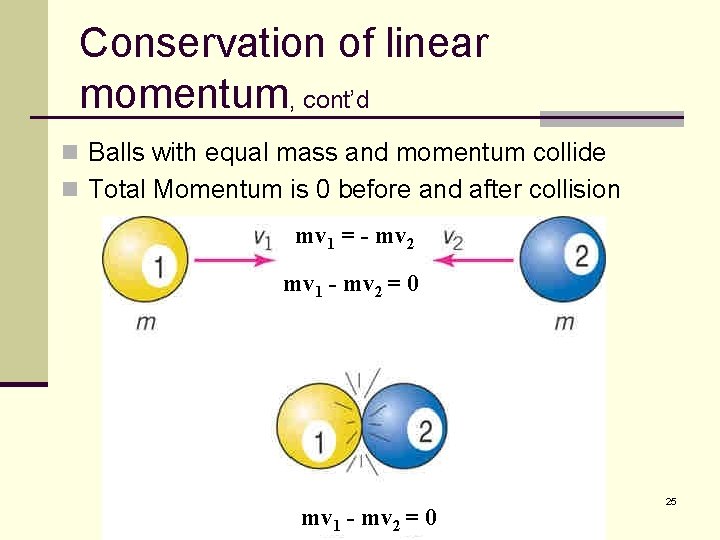
Conservation of linear momentum, cont’d n Balls with equal mass and momentum collide n Total Momentum is 0 before and after collision mv 1 = - mv 2 mv 1 - mv 2 = 0 25
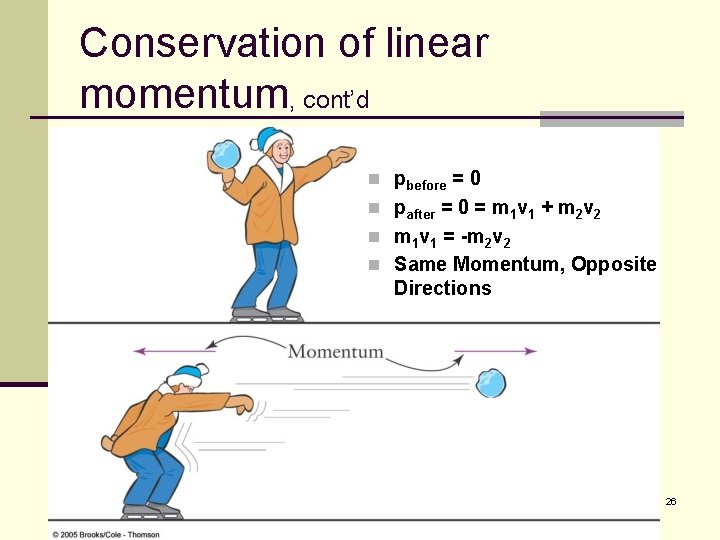
Conservation of linear momentum, cont’d n pbefore = 0 n pafter = 0 = m 1 v 1 + m 2 v 2 n m 1 v 1 = -m 2 v 2 n Same Momentum, Opposite Directions 26
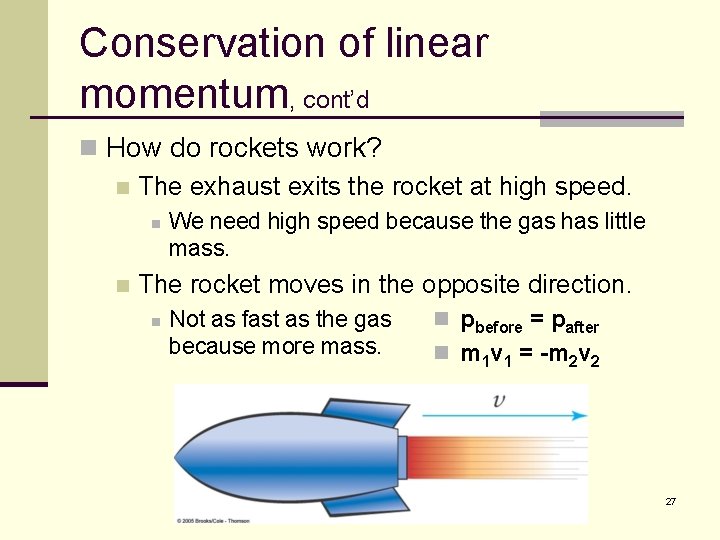
Conservation of linear momentum, cont’d n How do rockets work? n The exhaust exits the rocket at high speed. n n We need high speed because the gas has little mass. The rocket moves in the opposite direction. n Not as fast as the gas because more mass. n pbefore = pafter n m 1 v 1 = -m 2 v 2 27
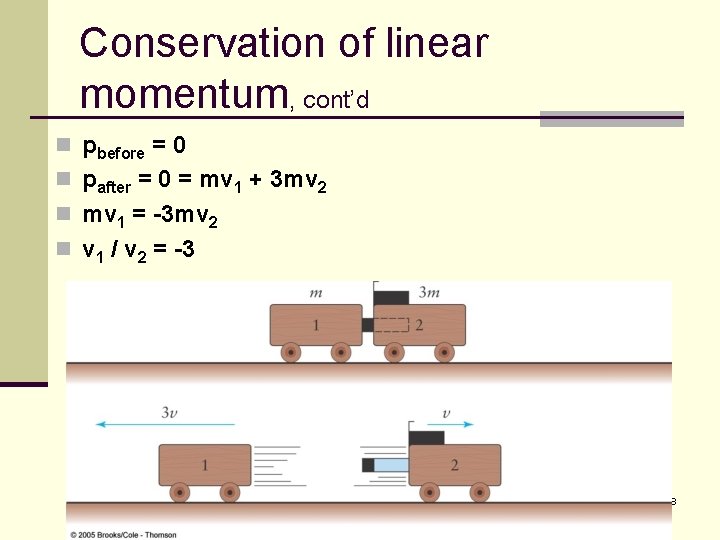
Conservation of linear momentum, cont’d n pbefore = 0 n pafter = 0 = mv 1 + 3 mv 2 n mv 1 = -3 mv 2 n v 1 / v 2 = -3 28
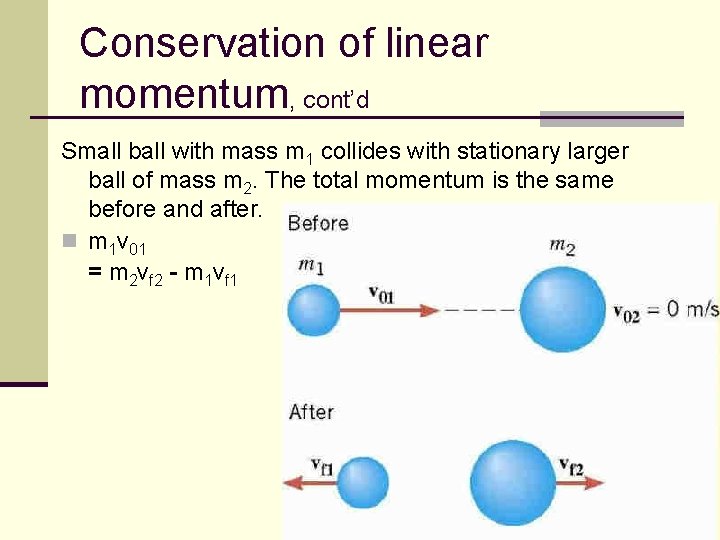
Conservation of linear momentum, cont’d Small ball with mass m 1 collides with stationary larger ball of mass m 2. The total momentum is the same before and after. n m 1 v 01 = m 2 vf 2 - m 1 vf 1 29
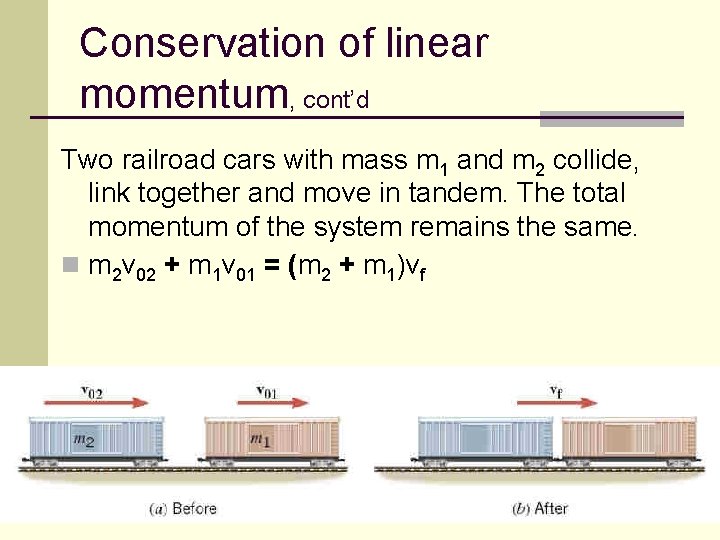
Conservation of linear momentum, cont’d Two railroad cars with mass m 1 and m 2 collide, link together and move in tandem. The total momentum of the system remains the same. n m 2 v 02 + m 1 v 01 = (m 2 + m 1)vf 30
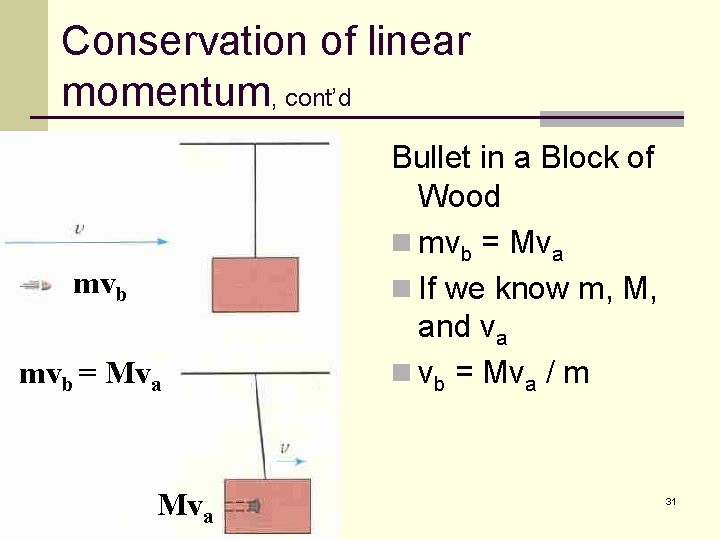
Conservation of linear momentum, cont’d mvb = Mva Bullet in a Block of Wood n mvb = Mva n If we know m, M, and va n vb = Mva / m 31
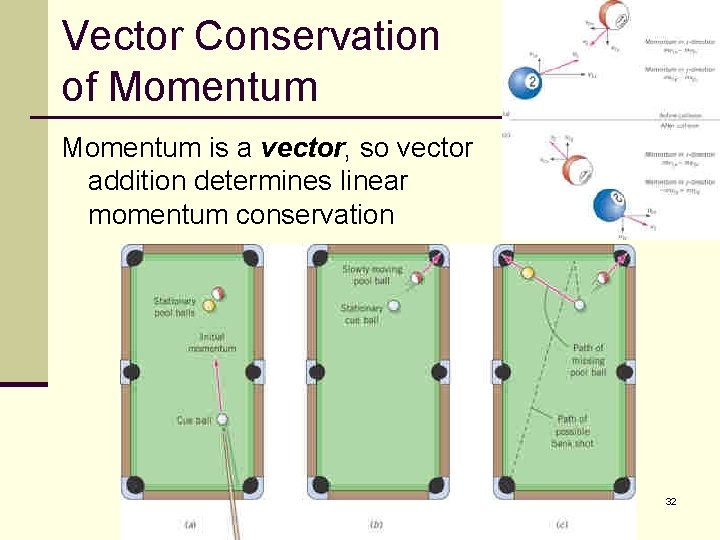
Vector Conservation of Momentum is a vector, so vector addition determines linear momentum conservation 32
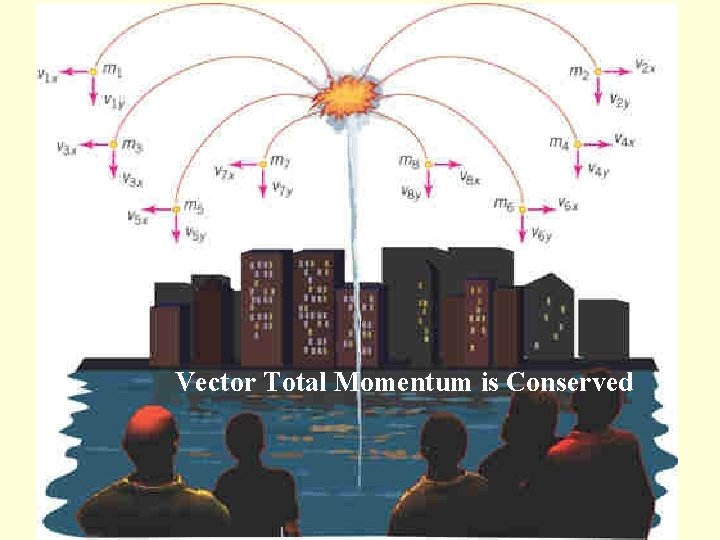
Vector Total Momentum is Conserved 33
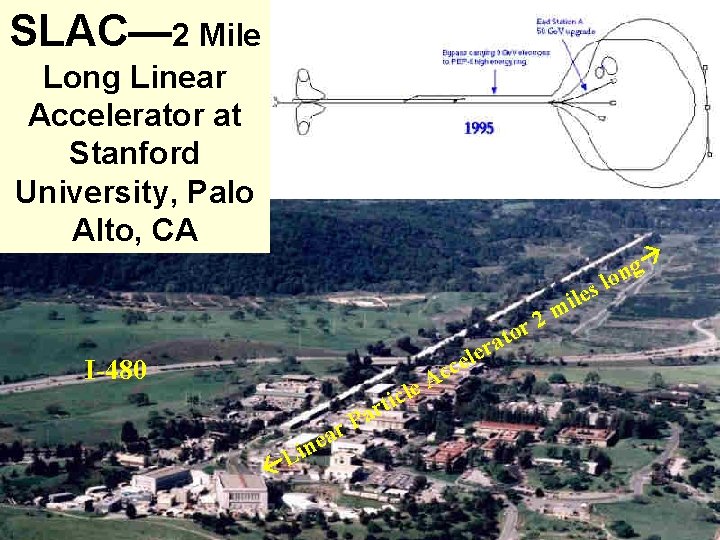
SLAC— 2 Mile Long Linear Accelerator at Stanford University, Palo Alto, CA a r e el I-480 a P r le c i rt 2 r to les i m g n lo c c A ea n i L 34
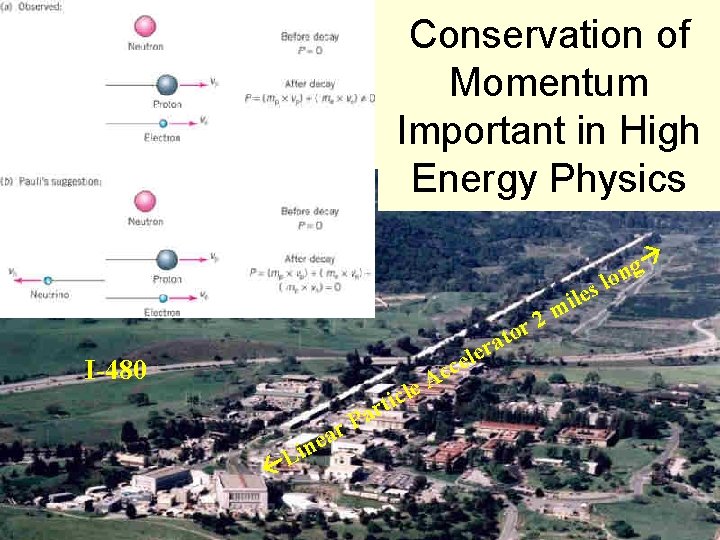
Conservation of Momentum Important in High Energy Physics a r e el I-480 a P r le c i rt 2 r to les i m g n lo c c A ea n i L 35
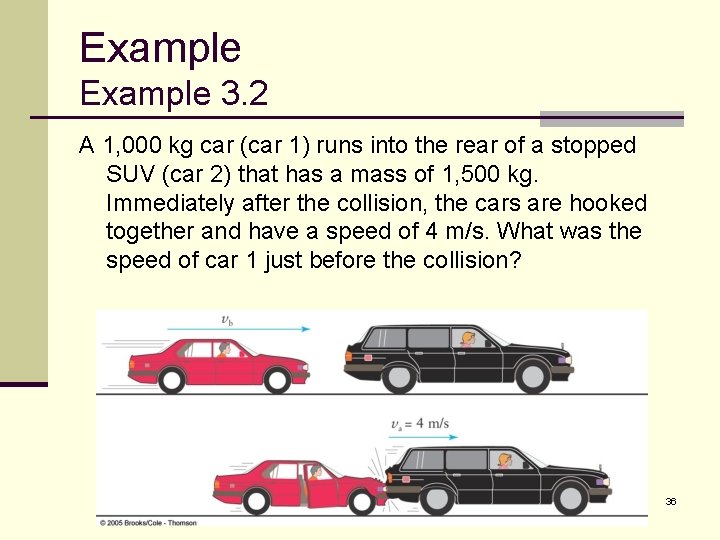
Example 3. 2 A 1, 000 kg car (car 1) runs into the rear of a stopped SUV (car 2) that has a mass of 1, 500 kg. Immediately after the collision, the cars are hooked together and have a speed of 4 m/s. What was the speed of car 1 just before the collision? 36
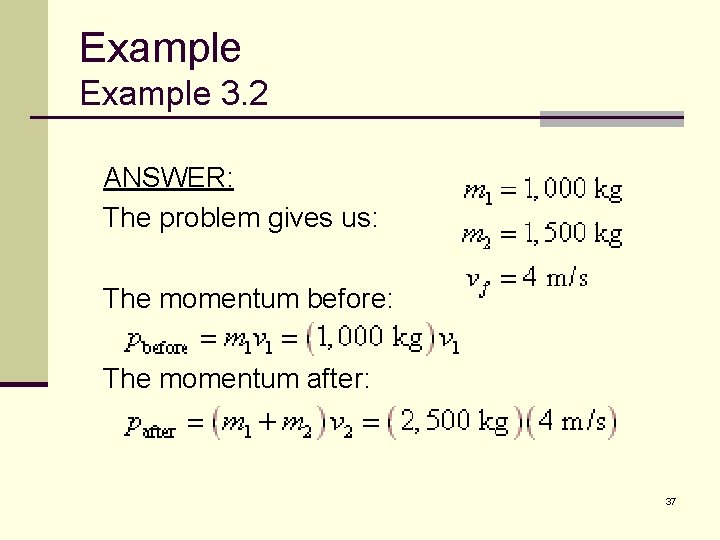
Example 3. 2 ANSWER: The problem gives us: The momentum before: The momentum after: 37
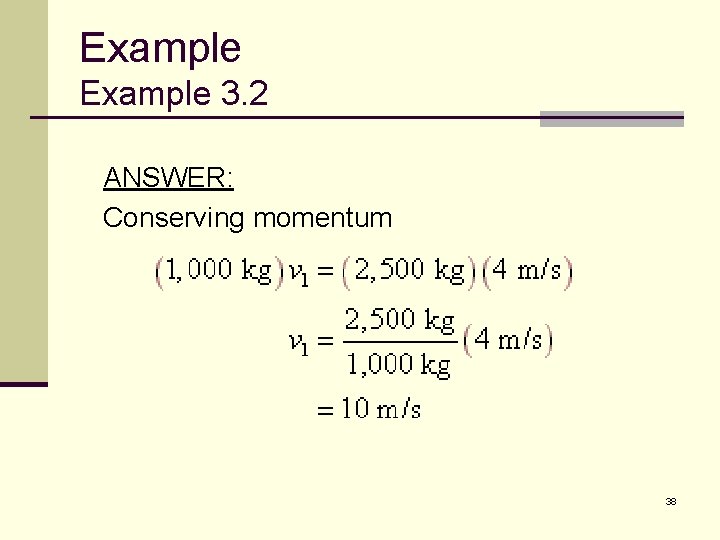
Example 3. 2 ANSWER: Conserving momentum 38
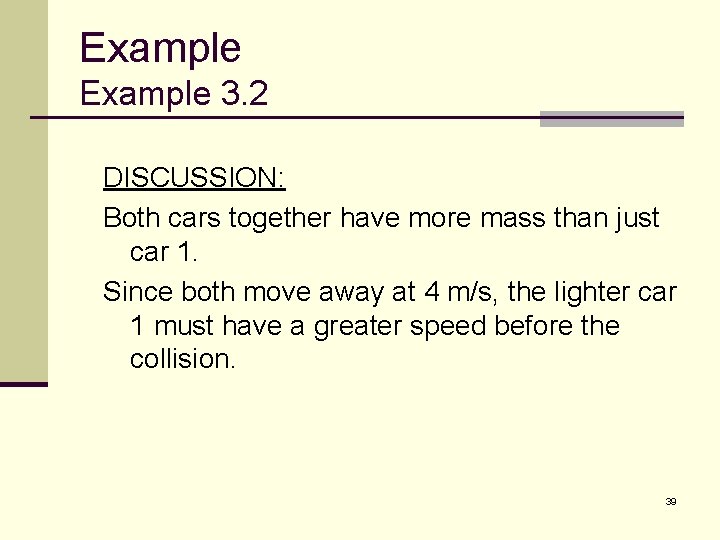
Example 3. 2 DISCUSSION: Both cars together have more mass than just car 1. Since both move away at 4 m/s, the lighter car 1 must have a greater speed before the collision. 39
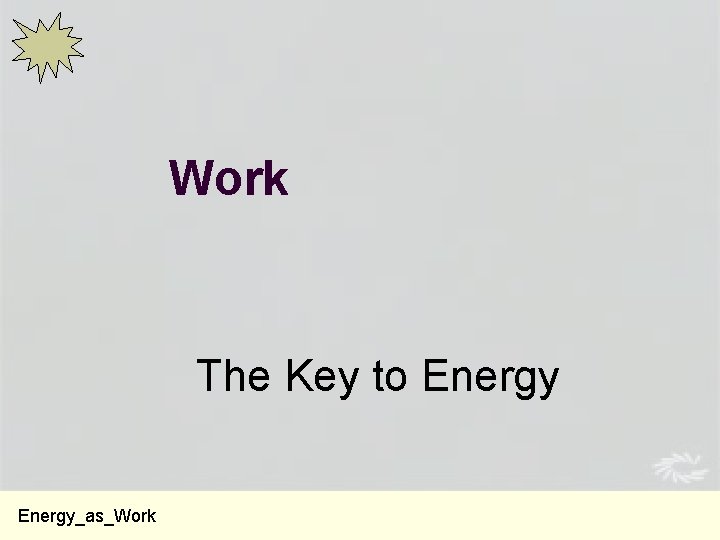
Work The Key to Energy_as_Work
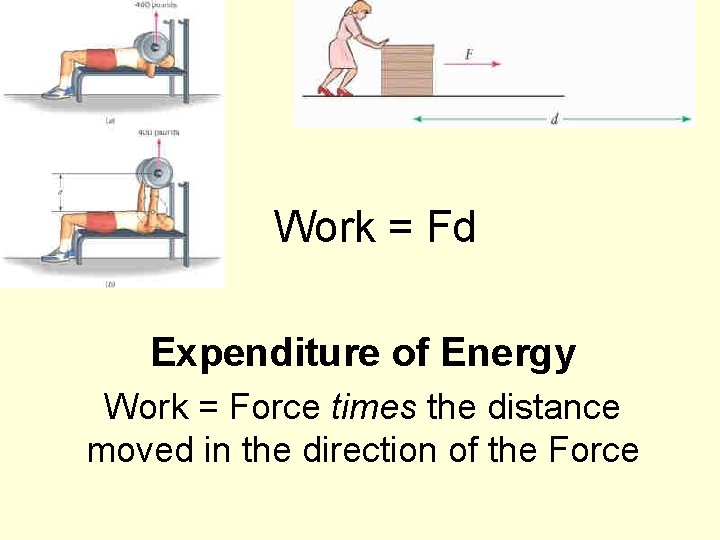
Work = Fd Expenditure of Energy Work = Force times the distance moved in the direction of the Force
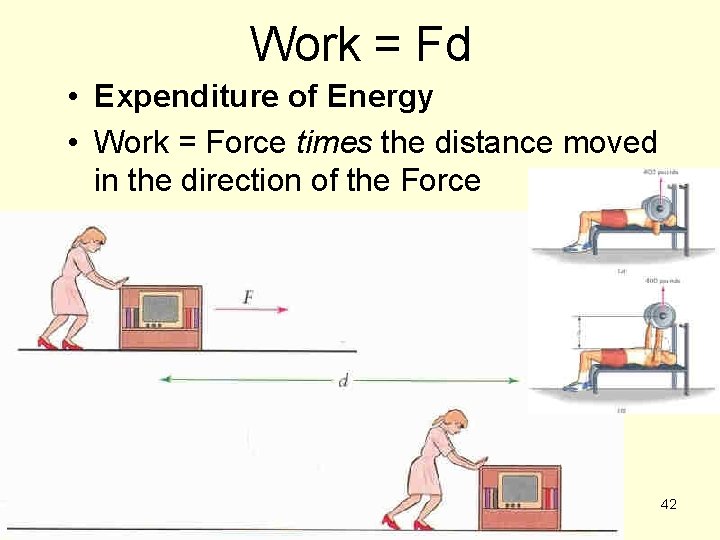
Work = Fd • Expenditure of Energy • Work = Force times the distance moved in the direction of the Force 42
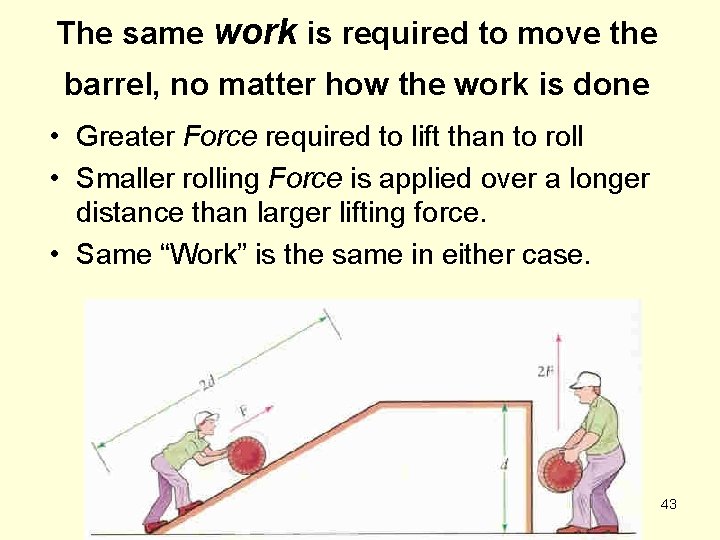
The same work is required to move the barrel, no matter how the work is done • Greater Force required to lift than to roll • Smaller rolling Force is applied over a longer distance than larger lifting force. • Same “Work” is the same in either case. 43
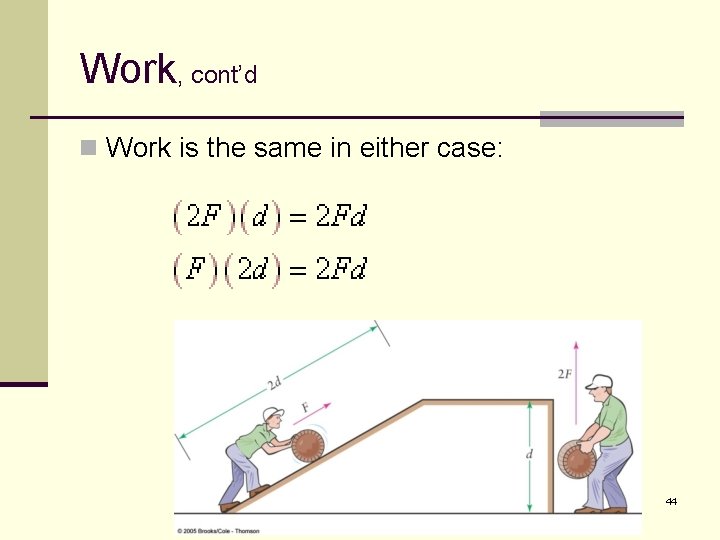
Work, cont’d n Work is the same in either case: 44
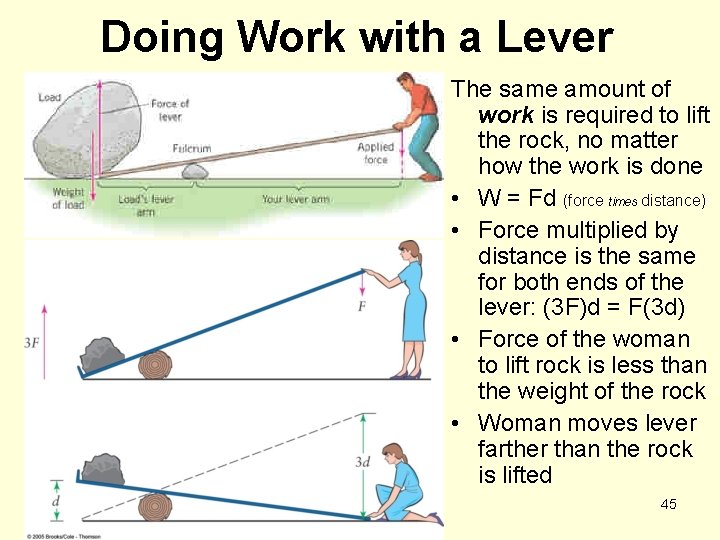
Doing Work with a Lever The same amount of work is required to lift the rock, no matter how the work is done • W = Fd (force times distance) • Force multiplied by distance is the same for both ends of the lever: (3 F)d = F(3 d) • Force of the woman to lift rock is less than the weight of the rock • Woman moves lever farther than the rock is lifted 45
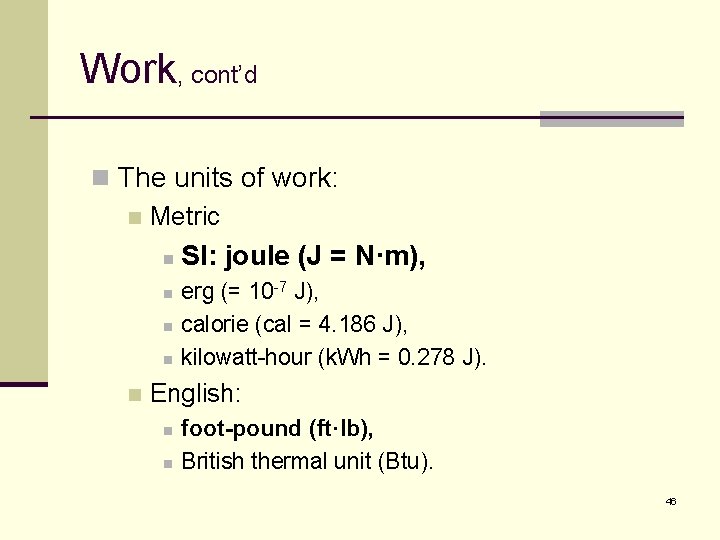
Work, cont’d n The units of work: n Metric n n n SI: joule (J = N·m), erg (= 10 -7 J), calorie (cal = 4. 186 J), kilowatt-hour (k. Wh = 0. 278 J). English: n n foot-pound (ft·lb), British thermal unit (Btu). 46
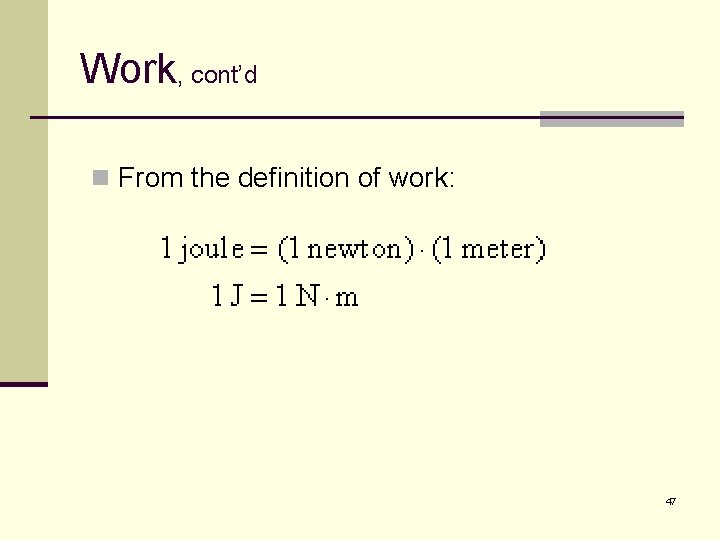
Work, cont’d n From the definition of work: 47
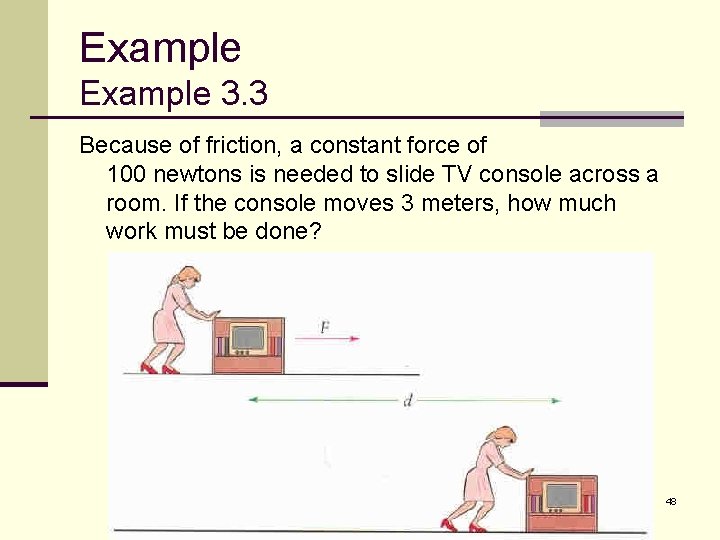
Example 3. 3 Because of friction, a constant force of 100 newtons is needed to slide TV console across a room. If the console moves 3 meters, how much work must be done? 48
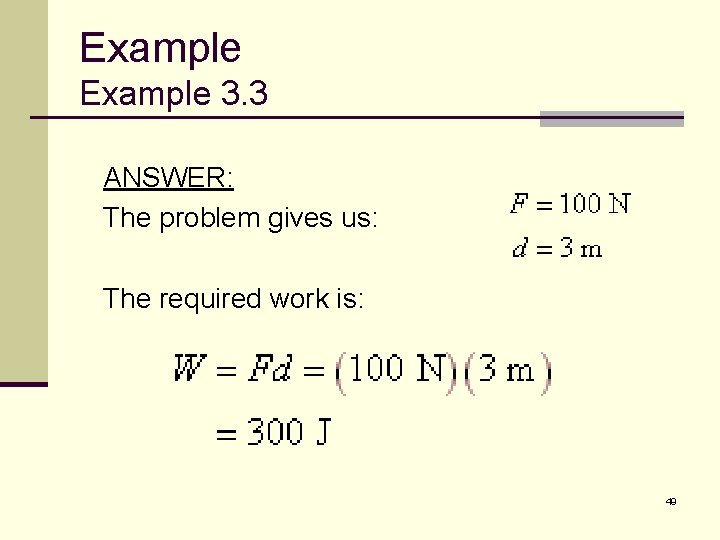
Example 3. 3 ANSWER: The problem gives us: The required work is: 49
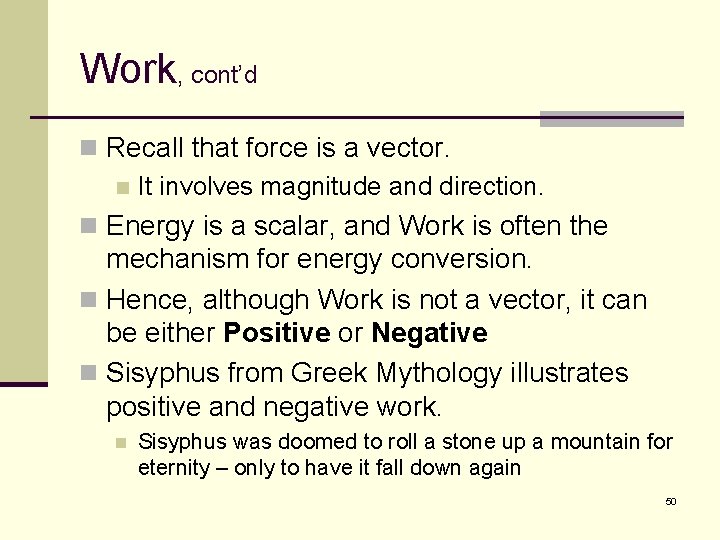
Work, cont’d n Recall that force is a vector. n It involves magnitude and direction. n Energy is a scalar, and Work is often the mechanism for energy conversion. n Hence, although Work is not a vector, it can be either Positive or Negative n Sisyphus from Greek Mythology illustrates positive and negative work. n Sisyphus was doomed to roll a stone up a mountain for eternity – only to have it fall down again 50
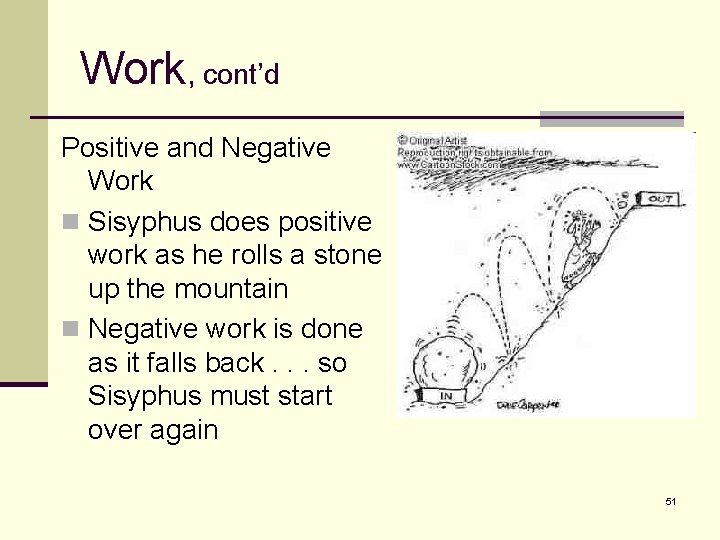
Work, cont’d Positive and Negative Work n Sisyphus does positive work as he rolls a stone up the mountain n Negative work is done as it falls back. . . so Sisyphus must start over again 51
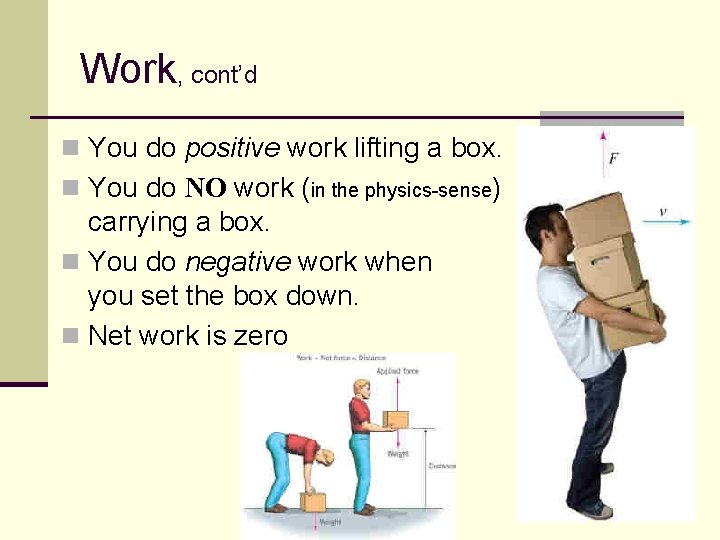
Work, cont’d n You do positive work lifting a box. n You do NO work (in the physics-sense) carrying a box. n You do negative work when you set the box down. n Net work is zero 52
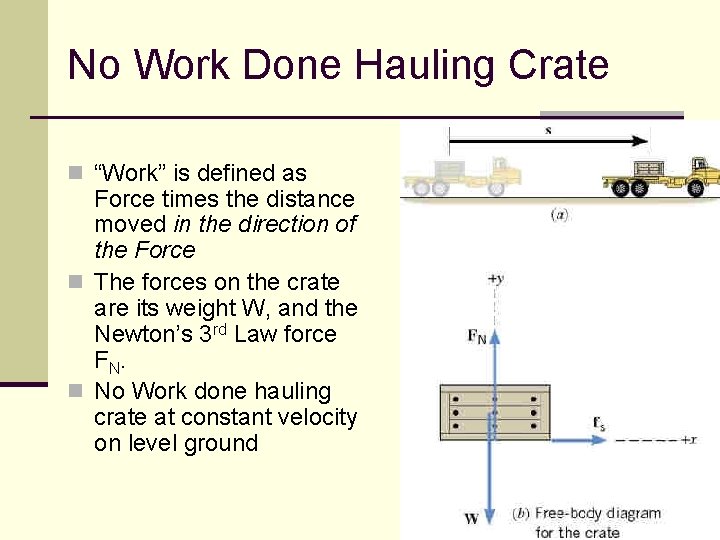
No Work Done Hauling Crate n “Work” is defined as Force times the distance moved in the direction of the Force n The forces on the crate are its weight W, and the Newton’s 3 rd Law force F N. n No Work done hauling crate at constant velocity on level ground 53
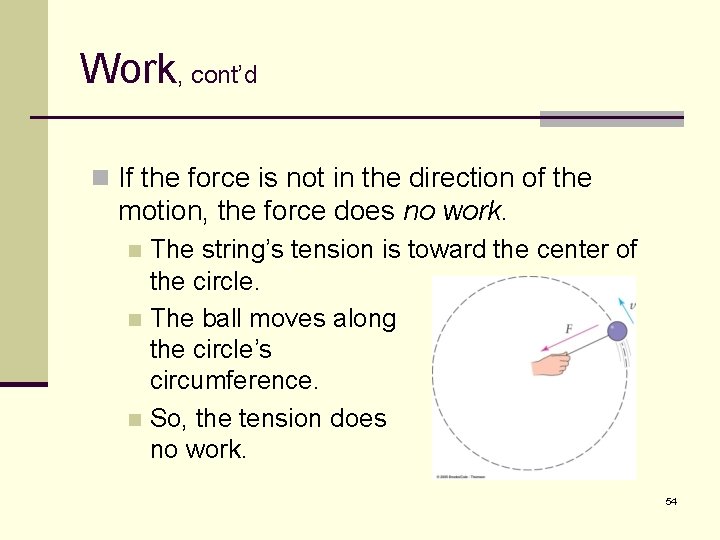
Work, cont’d n If the force is not in the direction of the motion, the force does no work. The string’s tension is toward the center of the circle. n The ball moves along the circle’s circumference. n So, the tension does no work. n 54
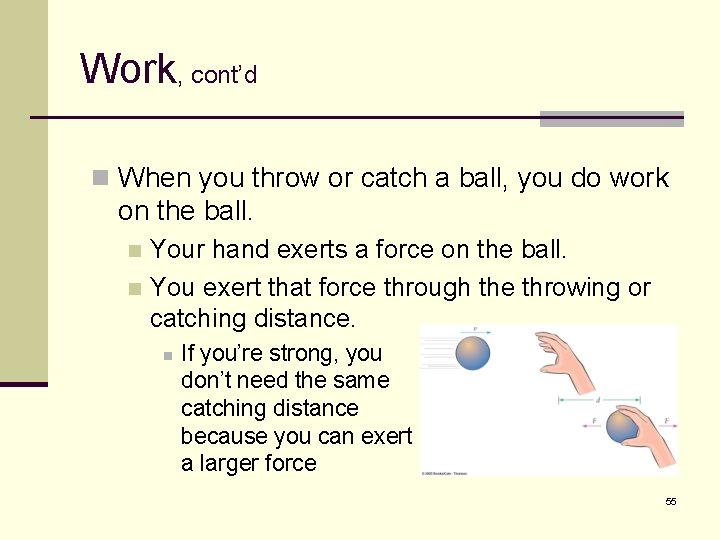
Work, cont’d n When you throw or catch a ball, you do work on the ball. Your hand exerts a force on the ball. n You exert that force through the throwing or catching distance. n n If you’re strong, you don’t need the same catching distance because you can exert a larger force 55
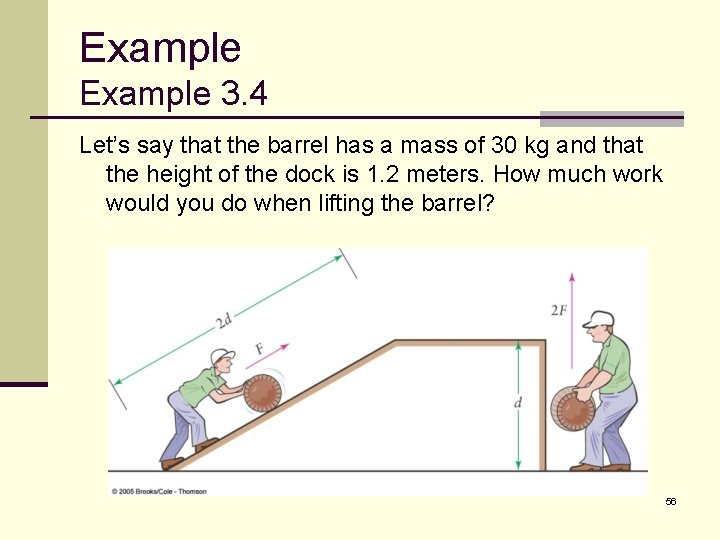
Example 3. 4 Let’s say that the barrel has a mass of 30 kg and that the height of the dock is 1. 2 meters. How much work would you do when lifting the barrel? 56
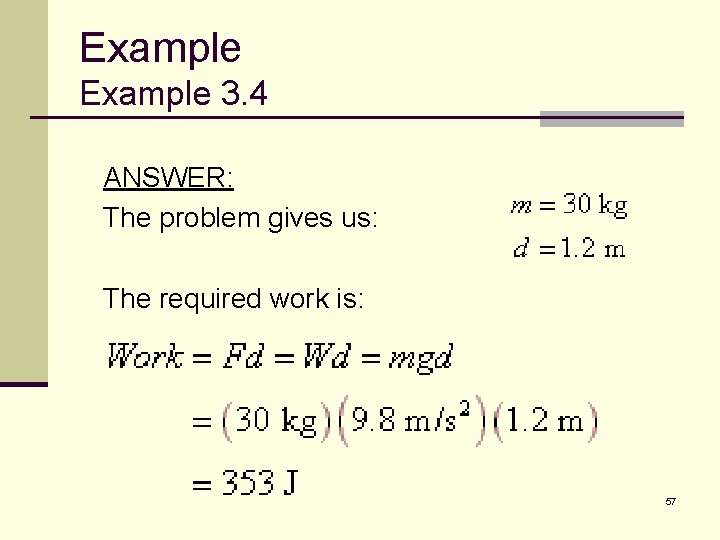
Example 3. 4 ANSWER: The problem gives us: The required work is: 57
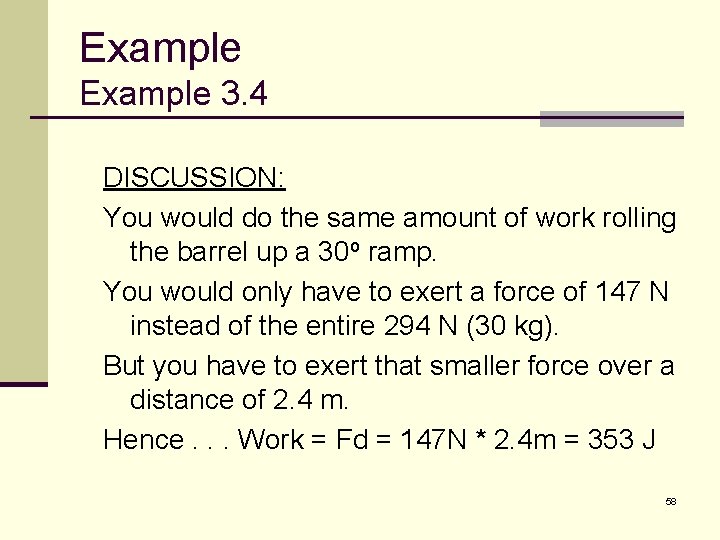
Example 3. 4 DISCUSSION: You would do the same amount of work rolling the barrel up a 30 o ramp. You would only have to exert a force of 147 N instead of the entire 294 N (30 kg). But you have to exert that smaller force over a distance of 2. 4 m. Hence. . . Work = Fd = 147 N * 2. 4 m = 353 J 58
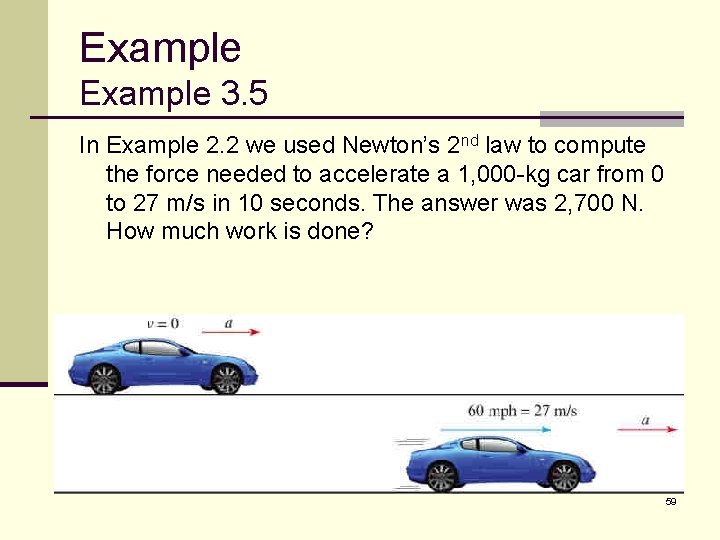
Example 3. 5 In Example 2. 2 we used Newton’s 2 nd law to compute the force needed to accelerate a 1, 000 -kg car from 0 to 27 m/s in 10 seconds. The answer was 2, 700 N. How much work is done? 59
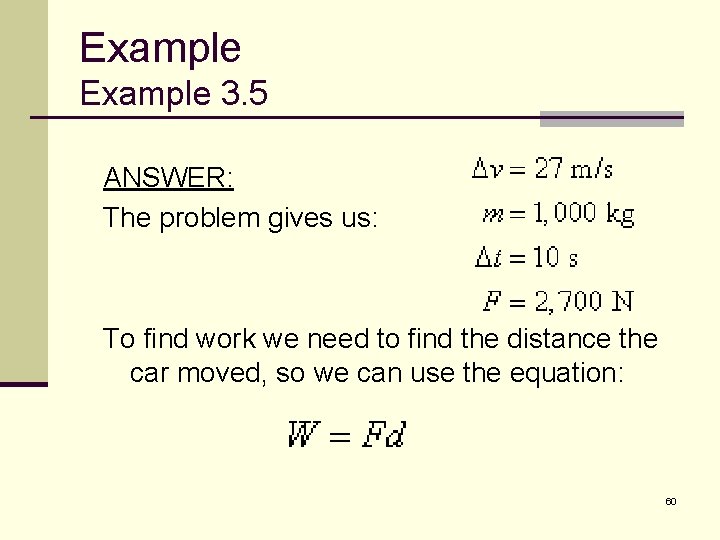
Example 3. 5 ANSWER: The problem gives us: To find work we need to find the distance the car moved, so we can use the equation: 60
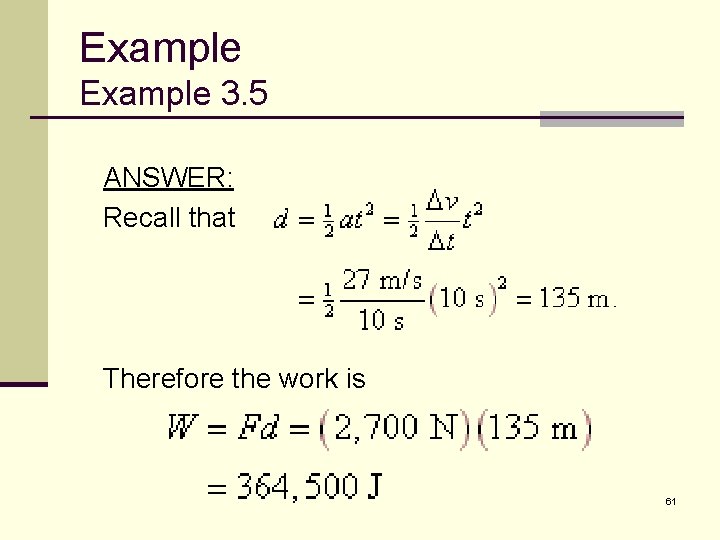
Example 3. 5 ANSWER: Recall that Therefore the work is 61
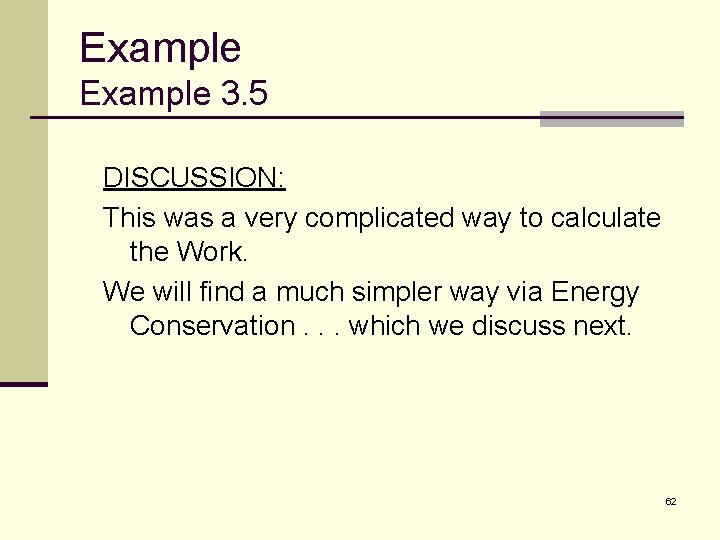
Example 3. 5 DISCUSSION: This was a very complicated way to calculate the Work. We will find a much simpler way via Energy Conservation. . . which we discuss next. 62
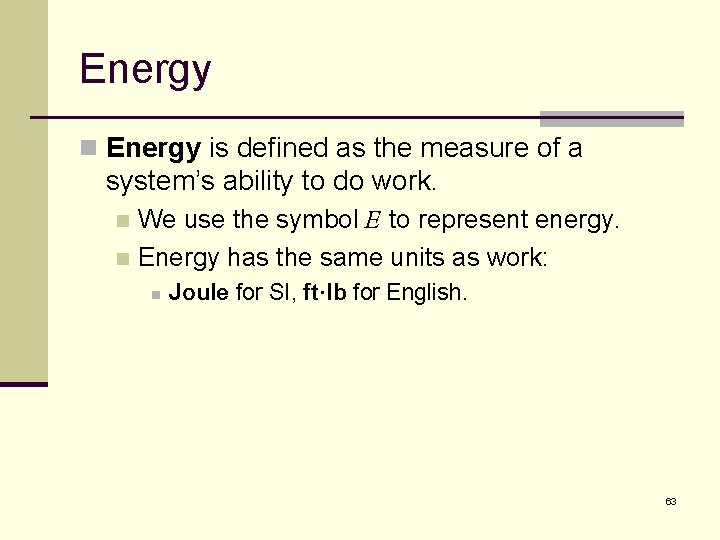
Energy n Energy is defined as the measure of a system’s ability to do work. We use the symbol E to represent energy. n Energy has the same units as work: n n Joule for SI, ft·lb for English. 63
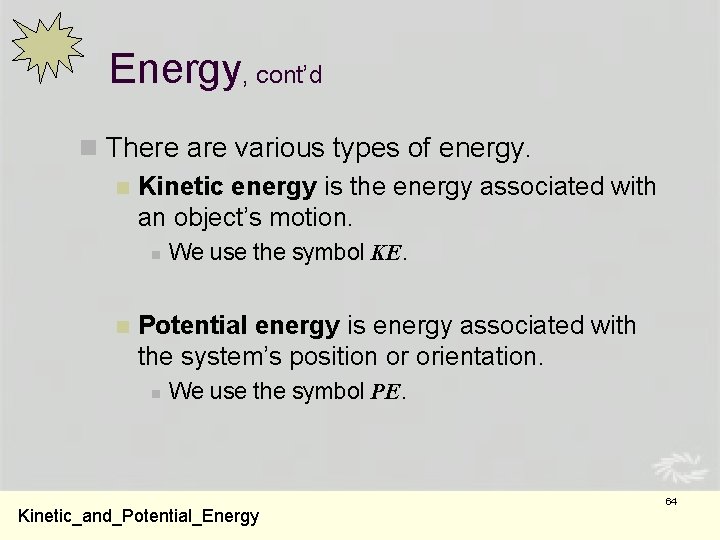
Energy, cont’d n There are various types of energy. n Kinetic energy is the energy associated with an object’s motion. n n We use the symbol KE. Potential energy is energy associated with the system’s position or orientation. n We use the symbol PE. Kinetic_and_Potential_Energy 64
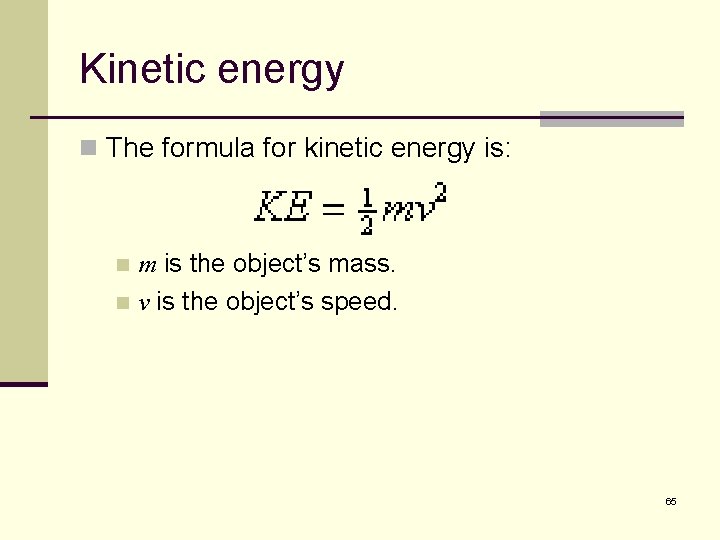
Kinetic energy n The formula for kinetic energy is: m is the object’s mass. n v is the object’s speed. n 65
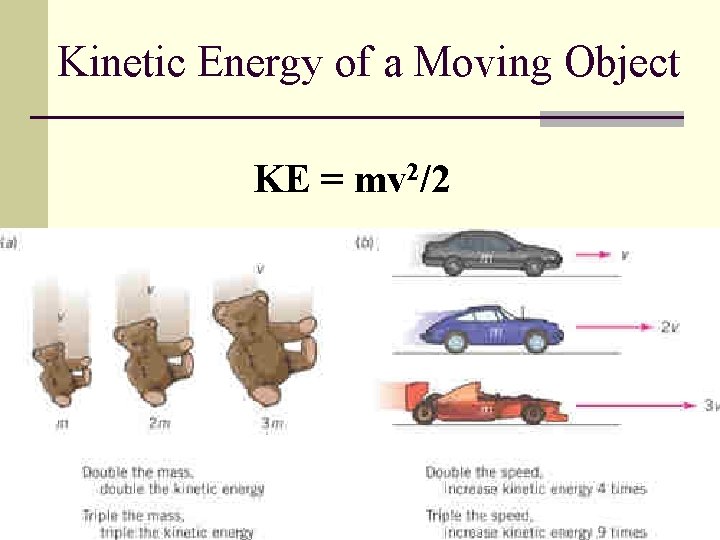
Kinetic Energy of a Moving Object KE = mv 2/2
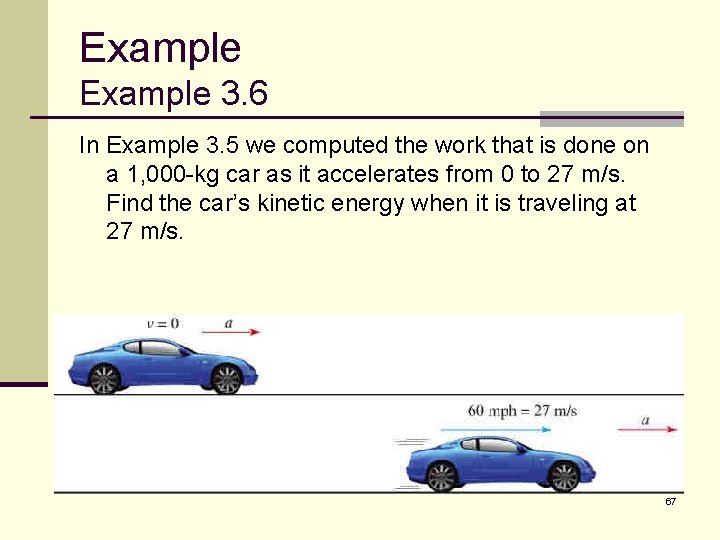
Example 3. 6 In Example 3. 5 we computed the work that is done on a 1, 000 -kg car as it accelerates from 0 to 27 m/s. Find the car’s kinetic energy when it is traveling at 27 m/s. 67
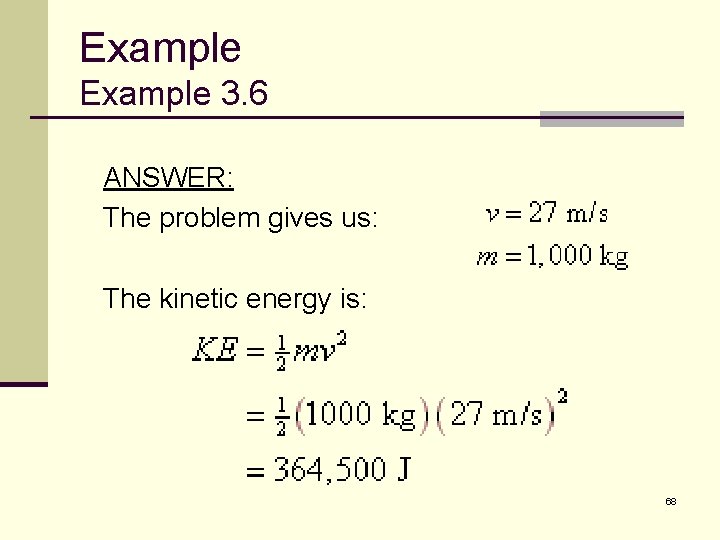
Example 3. 6 ANSWER: The problem gives us: The kinetic energy is: 68
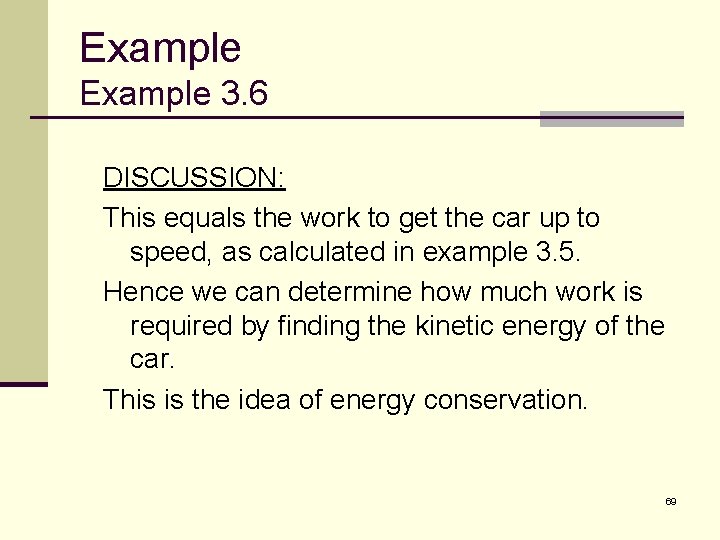
Example 3. 6 DISCUSSION: This equals the work to get the car up to speed, as calculated in example 3. 5. Hence we can determine how much work is required by finding the kinetic energy of the car. This is the idea of energy conservation. 69
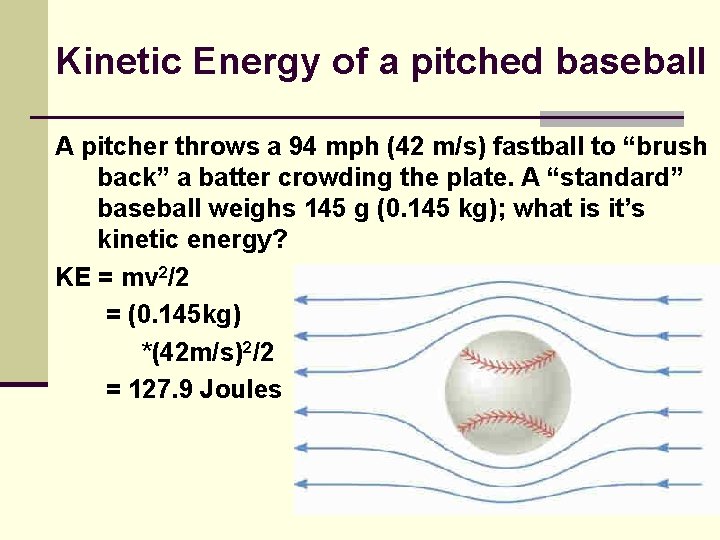
Kinetic Energy of a pitched baseball A pitcher throws a 94 mph (42 m/s) fastball to “brush back” a batter crowding the plate. A “standard” baseball weighs 145 g (0. 145 kg); what is it’s kinetic energy? KE = mv 2/2 = (0. 145 kg) *(42 m/s)2/2 = 127. 9 Joules 70
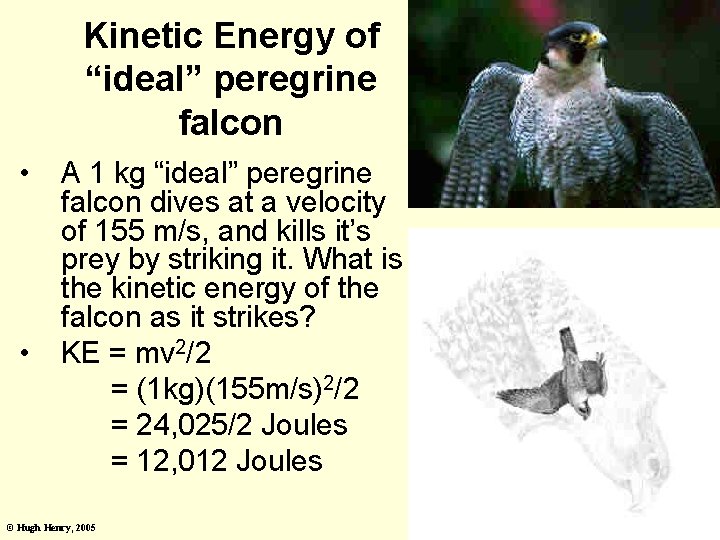
Kinetic Energy of “ideal” peregrine falcon • • A 1 kg “ideal” peregrine falcon dives at a velocity of 155 m/s, and kills it’s prey by striking it. What is the kinetic energy of the falcon as it strikes? KE = mv 2/2 = (1 kg)(155 m/s)2/2 = 24, 025/2 Joules = 12, 012 Joules 71 © Hugh Henry, 2005
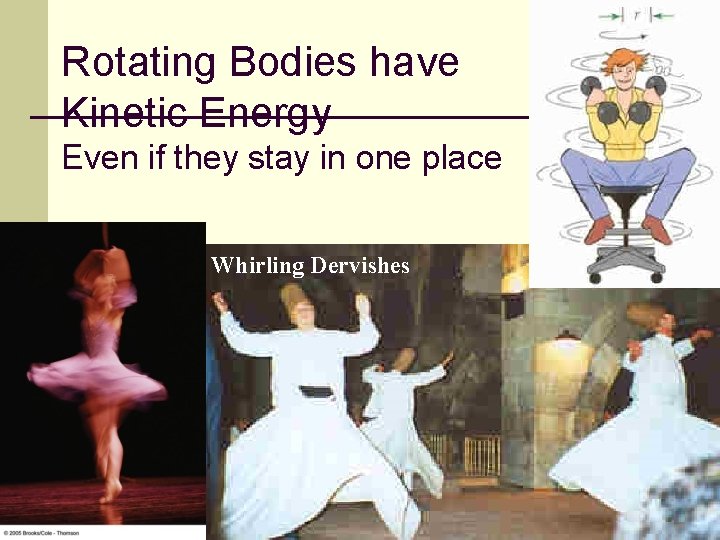
Rotating Bodies have Kinetic Energy Even if they stay in one place Whirling Dervishes 72
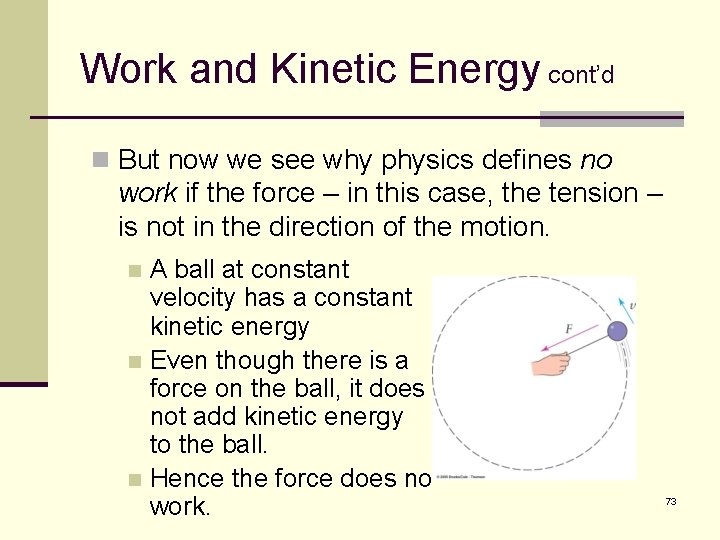
Work and Kinetic Energy cont’d n But now we see why physics defines no work if the force – in this case, the tension – is not in the direction of the motion. A ball at constant velocity has a constant kinetic energy n Even though there is a force on the ball, it does not add kinetic energy to the ball. n Hence the force does no work. n 73
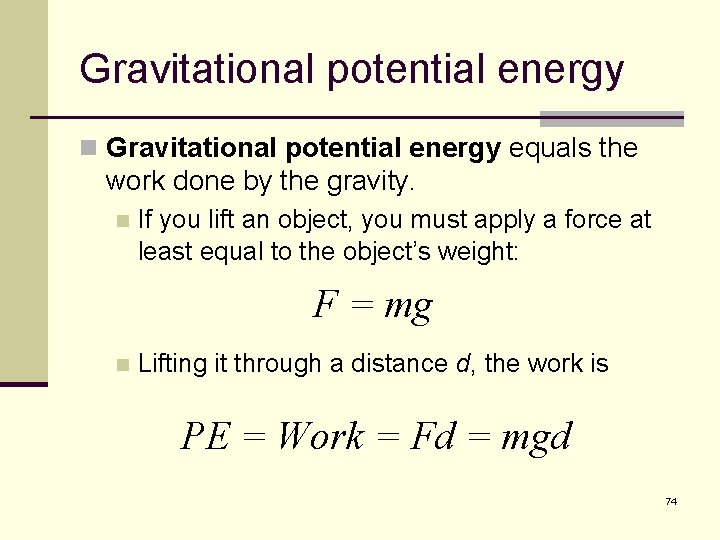
Gravitational potential energy n Gravitational potential energy equals the work done by the gravity. n If you lift an object, you must apply a force at least equal to the object’s weight: F = mg n Lifting it through a distance d, the work is PE = Work = Fd = mgd 74
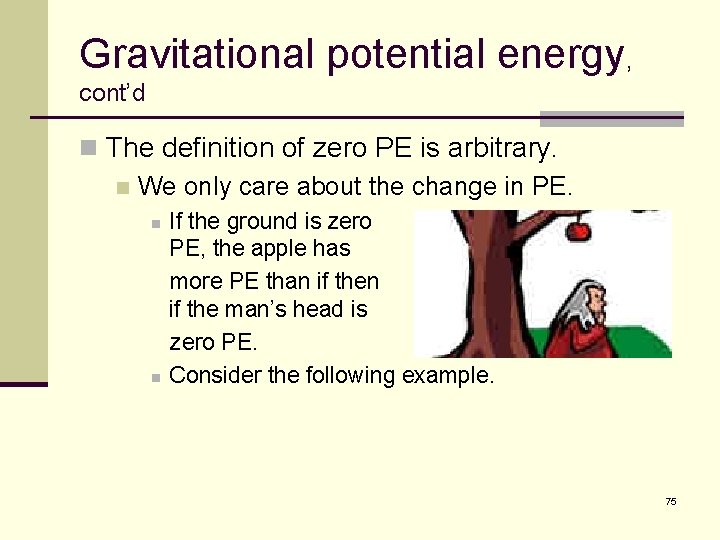
Gravitational potential energy, cont’d n The definition of zero PE is arbitrary. n We only care about the change in PE. n n If the ground is zero PE, the apple has more PE than if the man’s head is zero PE. Consider the following example. 75
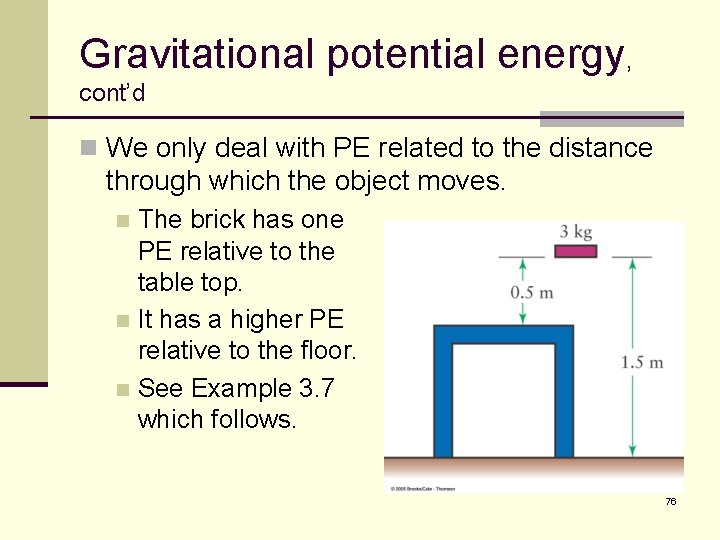
Gravitational potential energy, cont’d n We only deal with PE related to the distance through which the object moves. The brick has one PE relative to the table top. n It has a higher PE relative to the floor. n See Example 3. 7 which follows. n 76
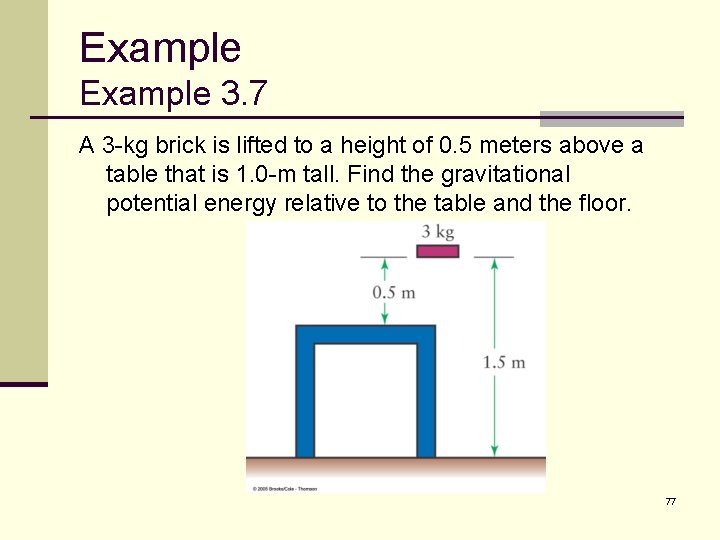
Example 3. 7 A 3 -kg brick is lifted to a height of 0. 5 meters above a table that is 1. 0 -m tall. Find the gravitational potential energy relative to the table and the floor. 77
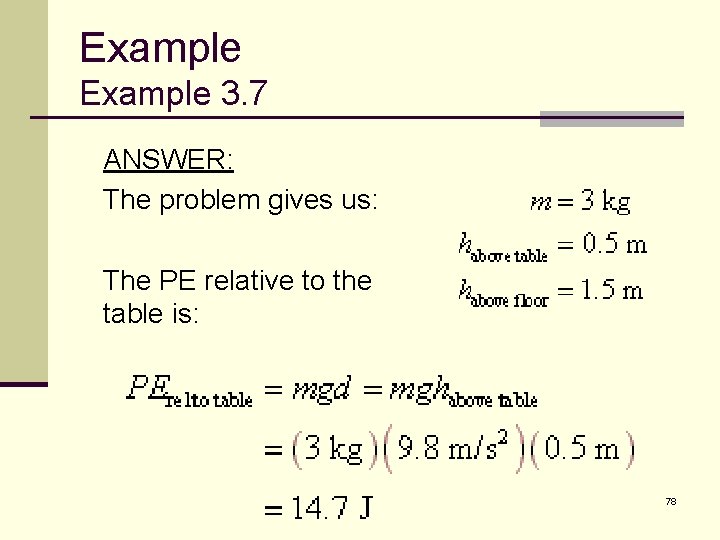
Example 3. 7 ANSWER: The problem gives us: The PE relative to the table is: 78
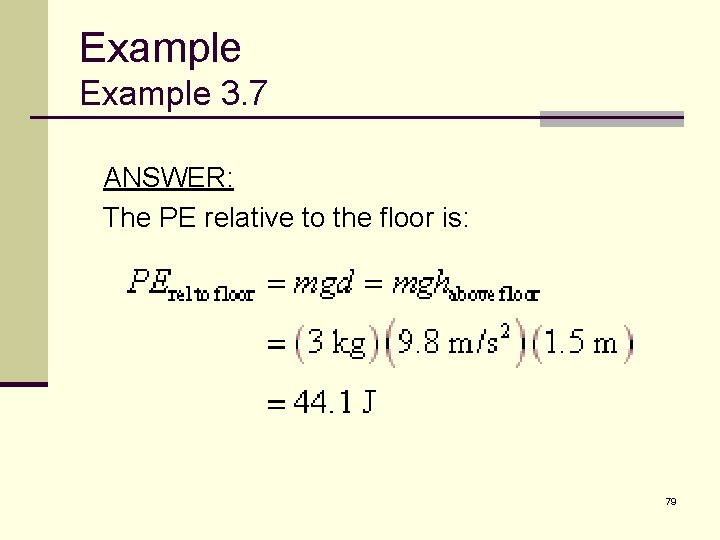
Example 3. 7 ANSWER: The PE relative to the floor is: 79
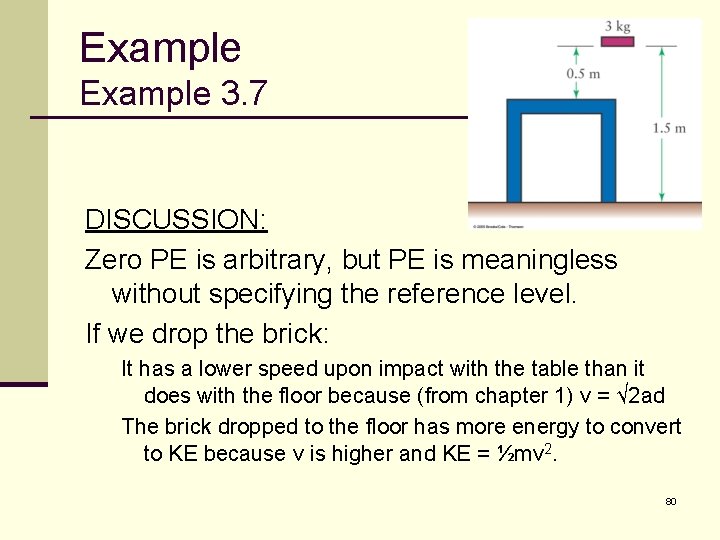
Example 3. 7 DISCUSSION: Zero PE is arbitrary, but PE is meaningless without specifying the reference level. If we drop the brick: It has a lower speed upon impact with the table than it does with the floor because (from chapter 1) v = √ 2 ad The brick dropped to the floor has more energy to convert to KE because v is higher and KE = ½mv 2. 80
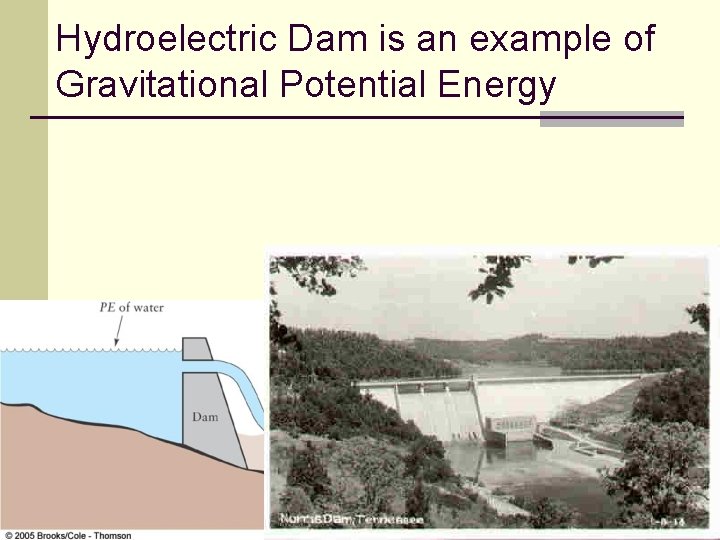
Hydroelectric Dam is an example of Gravitational Potential Energy 81
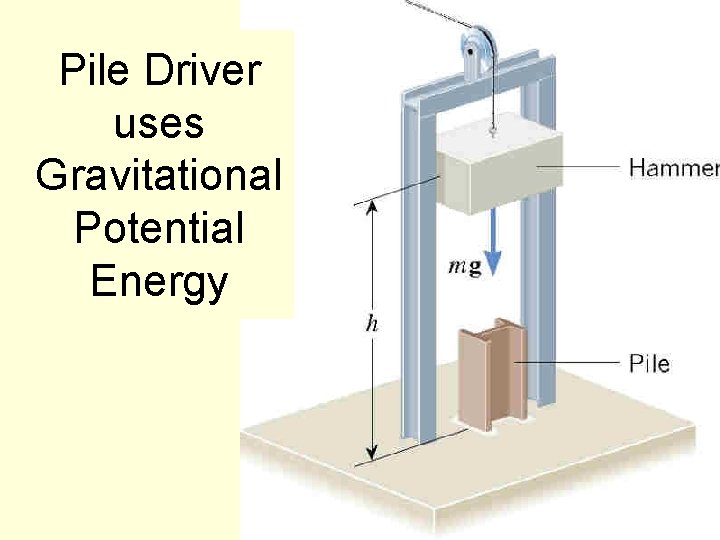
Pile Driver uses Gravitational Potential Energy 82
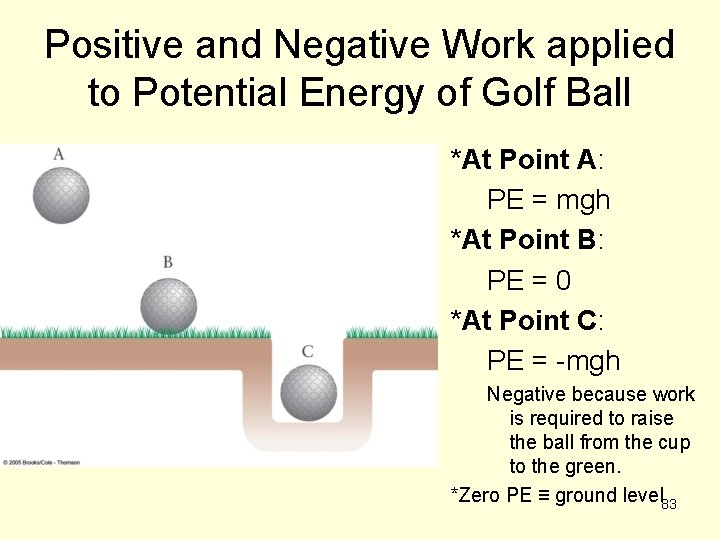
Positive and Negative Work applied to Potential Energy of Golf Ball *At Point A: PE = mgh *At Point B: PE = 0 *At Point C: PE = -mgh Negative because work is required to raise the ball from the cup to the green. *Zero PE ≡ ground level 83
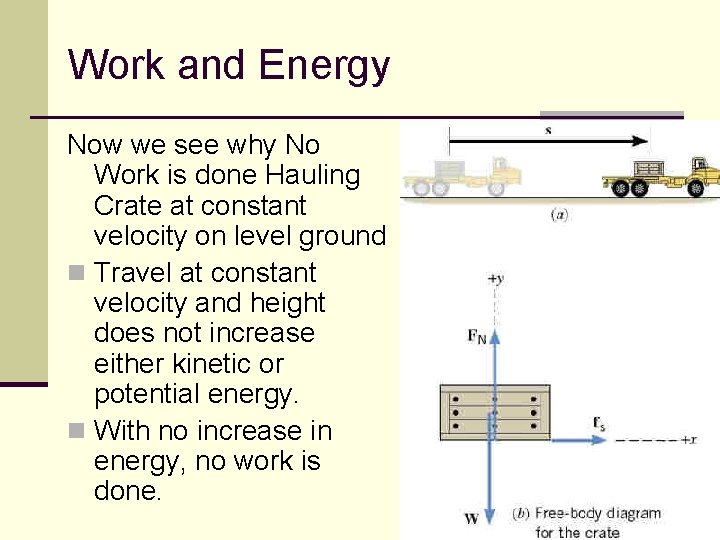
Work and Energy Now we see why No Work is done Hauling Crate at constant velocity on level ground n Travel at constant velocity and height does not increase either kinetic or potential energy. n With no increase in energy, no work is done. 84
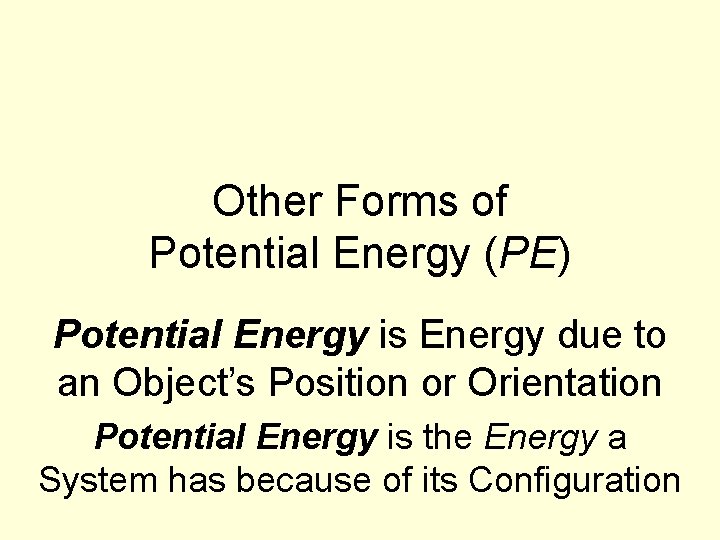
Other Forms of Potential Energy (PE) Potential Energy is Energy due to an Object’s Position or Orientation Potential Energy is the Energy a System has because of its Configuration
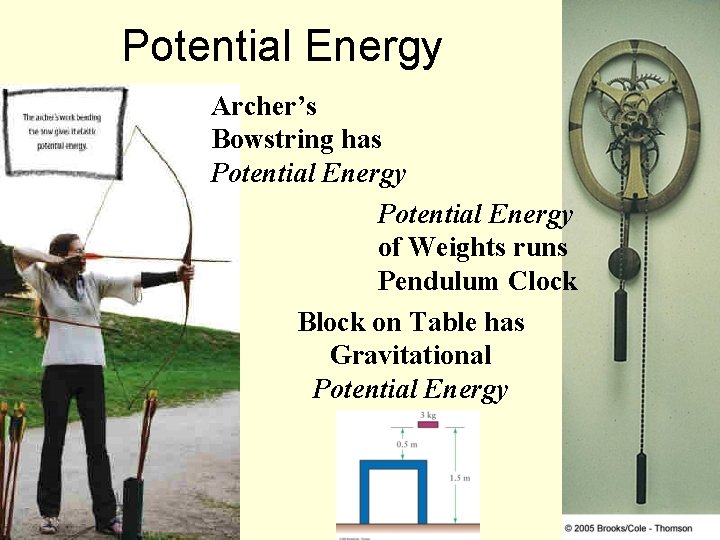
Potential Energy Archer’s Bowstring has Potential Energy of Weights runs Pendulum Clock Block on Table has Gravitational Potential Energy 86
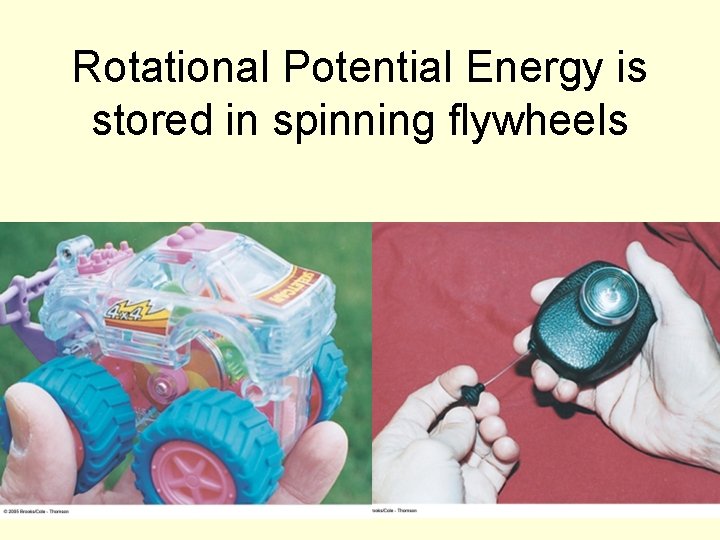
Rotational Potential Energy is stored in spinning flywheels 87
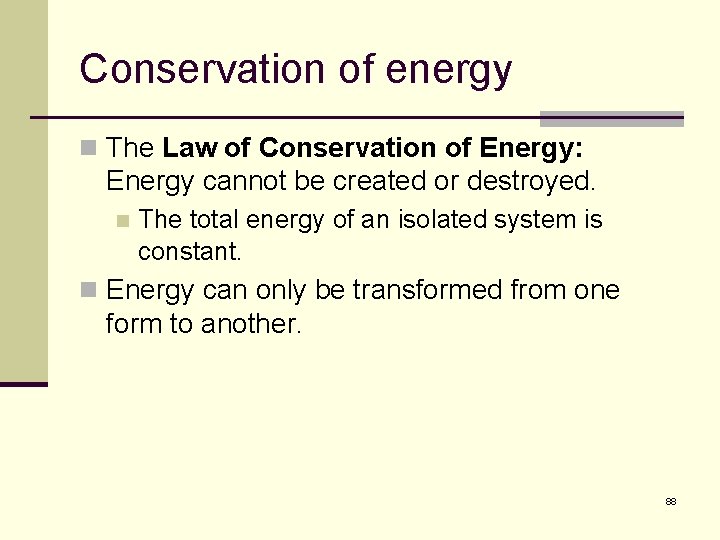
Conservation of energy n The Law of Conservation of Energy: Energy cannot be created or destroyed. n The total energy of an isolated system is constant. n Energy can only be transformed from one form to another. 88
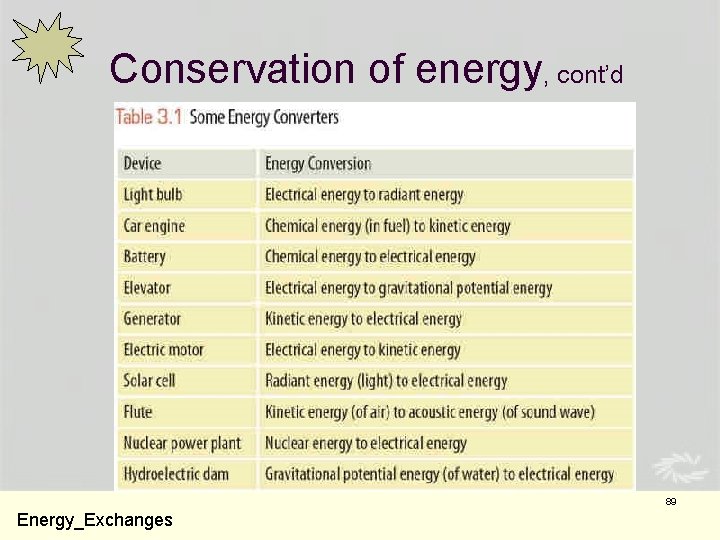
Conservation of energy, cont’d 89 Energy_Exchanges
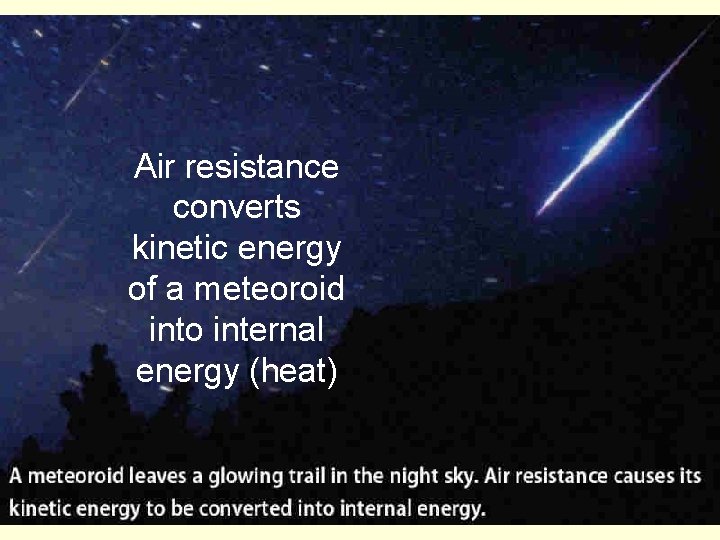
Air resistance converts kinetic energy of a meteoroid into internal energy (heat)
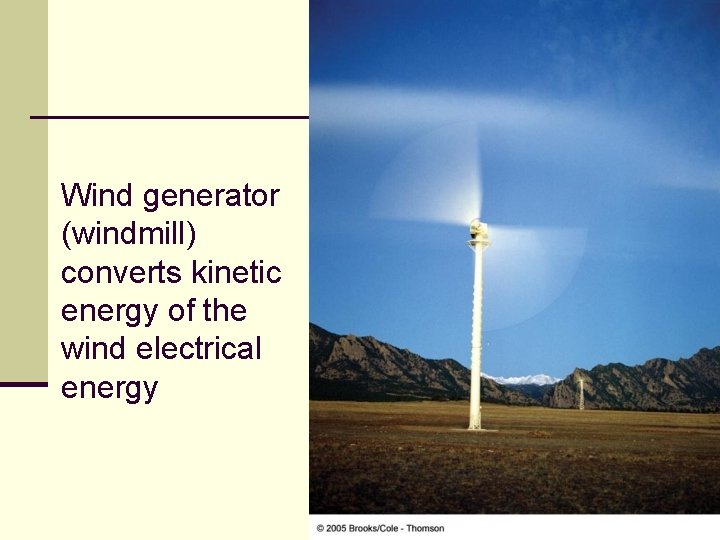
Wind generator (windmill) converts kinetic energy of the wind electrical energy 91
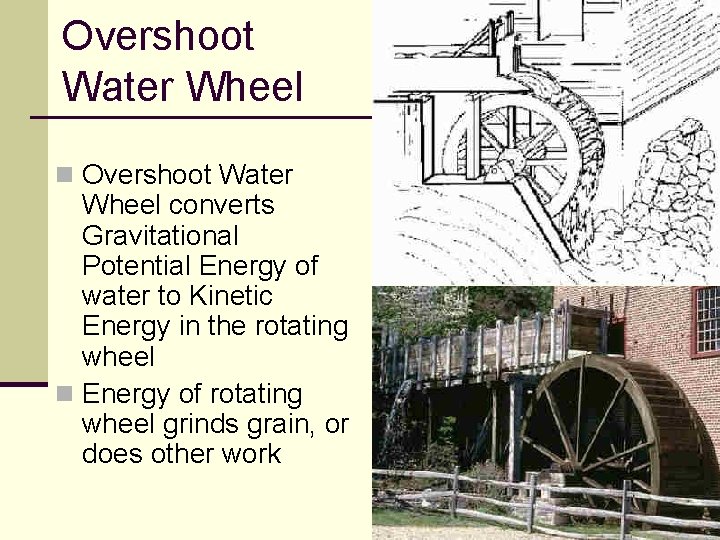
Overshoot Water Wheel n Overshoot Water Wheel converts Gravitational Potential Energy of water to Kinetic Energy in the rotating wheel n Energy of rotating wheel grinds grain, or does other work 92
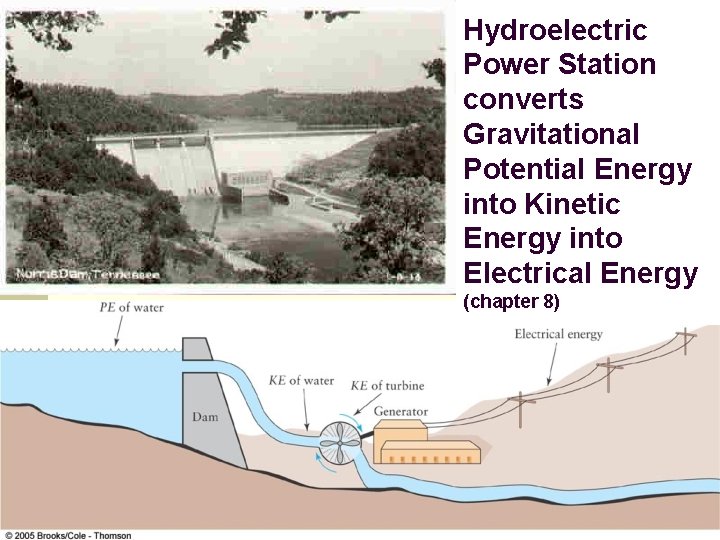
Hydroelectric Power Station converts Gravitational Potential Energy into Kinetic Energy into Electrical Energy (chapter 8) 93
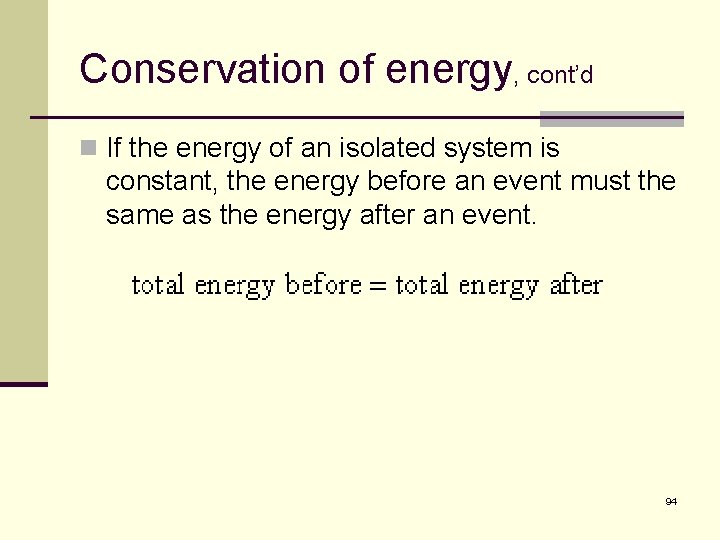
Conservation of energy, cont’d n If the energy of an isolated system is constant, the energy before an event must the same as the energy after an event. 94
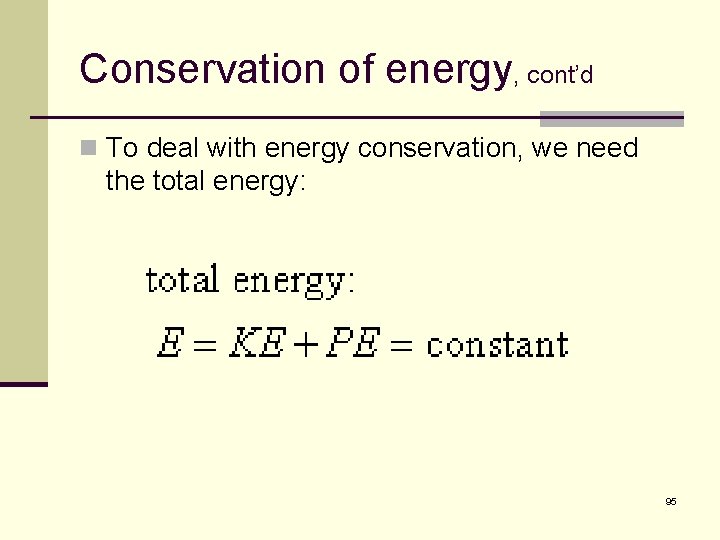
Conservation of energy, cont’d n To deal with energy conservation, we need the total energy: 95
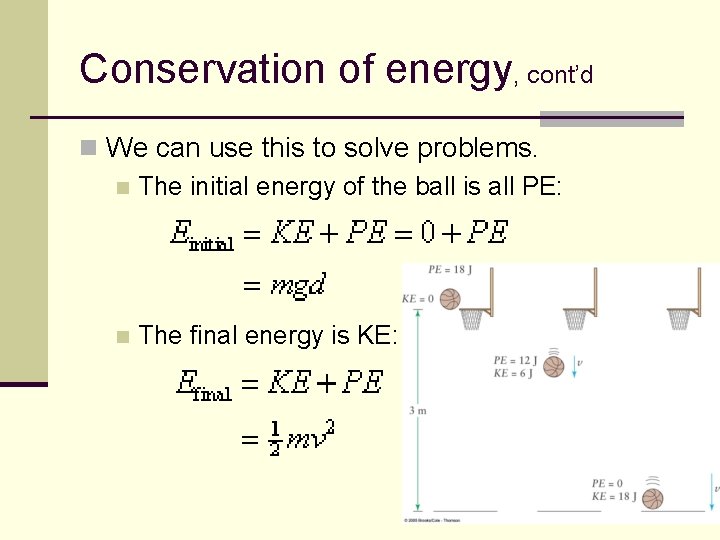
Conservation of energy, cont’d n We can use this to solve problems. n The initial energy of the ball is all PE: n The final energy is KE: 96
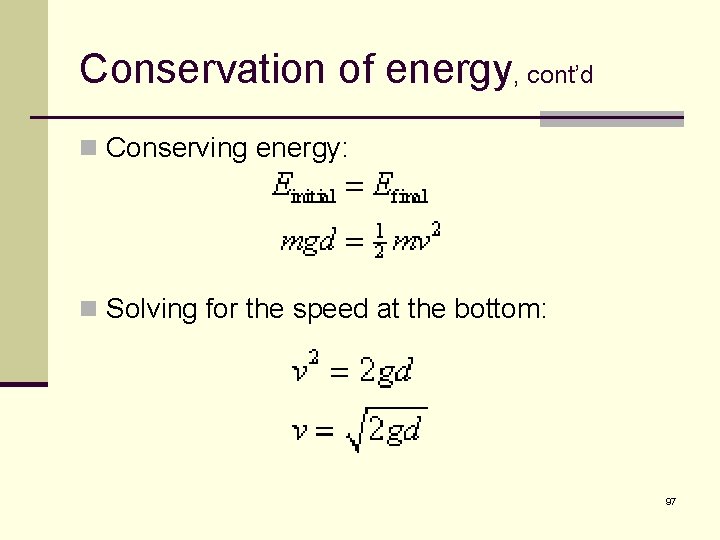
Conservation of energy, cont’d n Conserving energy: n Solving for the speed at the bottom: 97
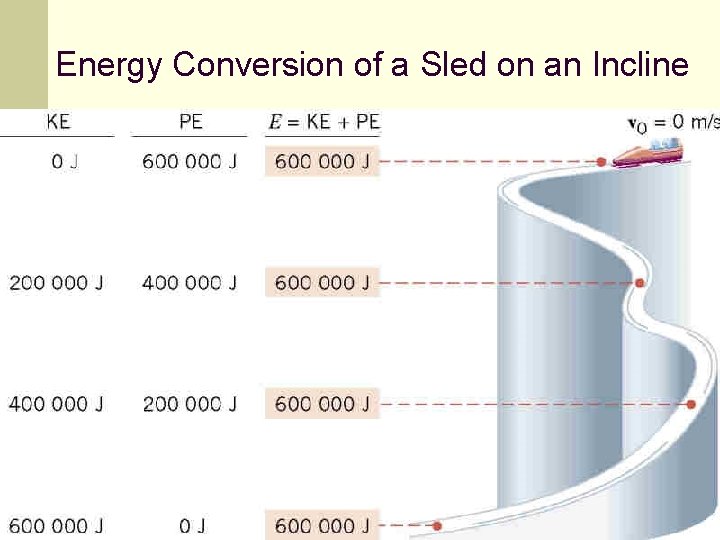
Energy Conversion of a Sled on an Incline 98
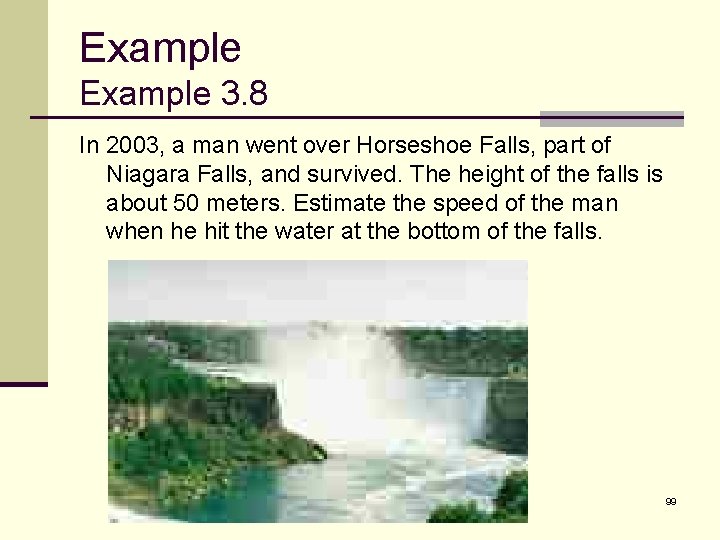
Example 3. 8 In 2003, a man went over Horseshoe Falls, part of Niagara Falls, and survived. The height of the falls is about 50 meters. Estimate the speed of the man when he hit the water at the bottom of the falls. 99
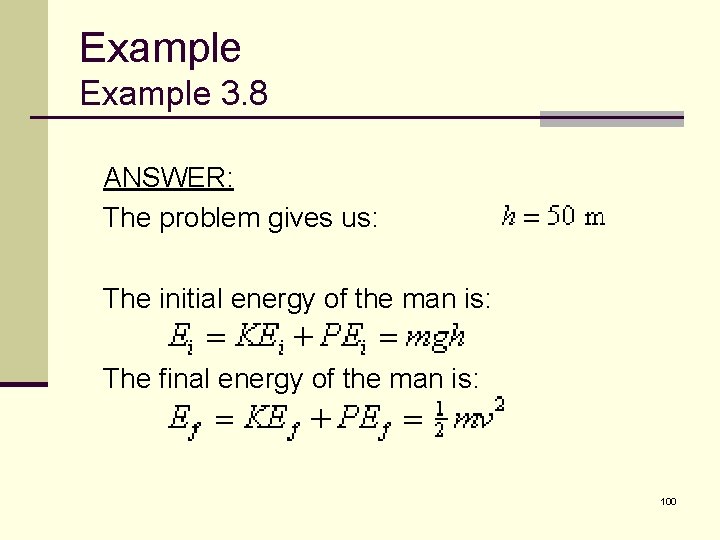
Example 3. 8 ANSWER: The problem gives us: The initial energy of the man is: The final energy of the man is: 100
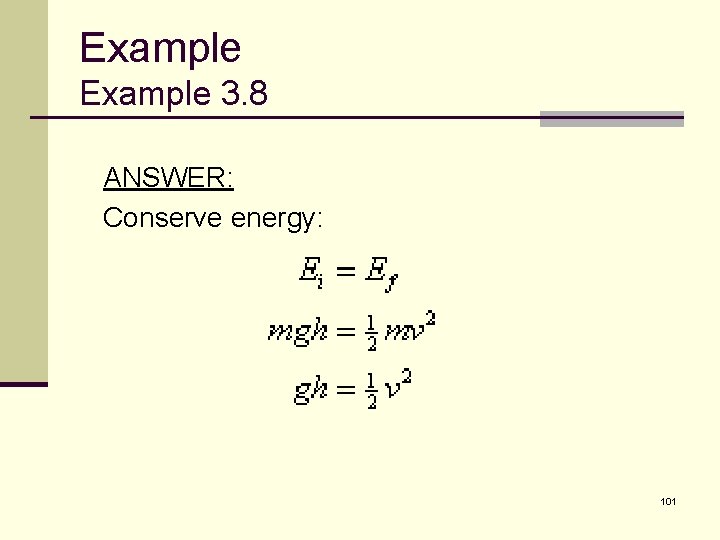
Example 3. 8 ANSWER: Conserve energy: 101
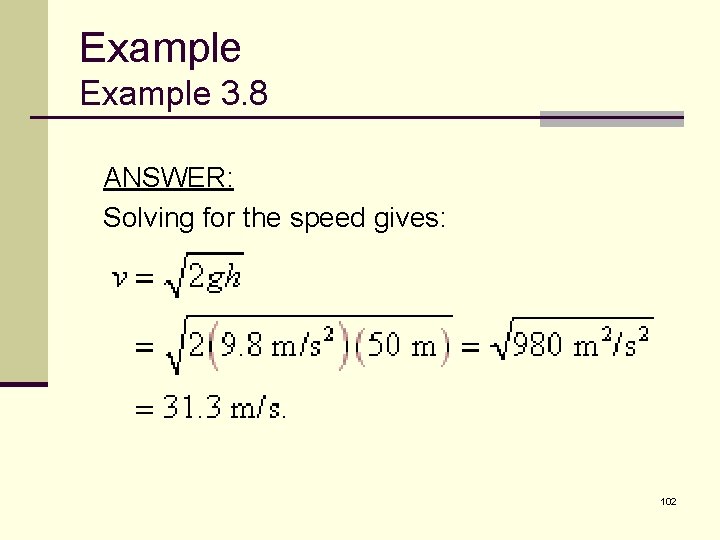
Example 3. 8 ANSWER: Solving for the speed gives: 102
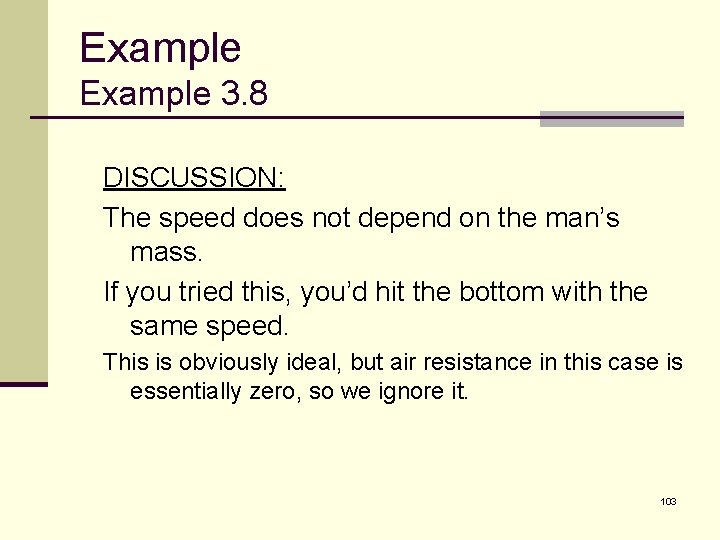
Example 3. 8 DISCUSSION: The speed does not depend on the man’s mass. If you tried this, you’d hit the bottom with the same speed. This is obviously ideal, but air resistance in this case is essentially zero, so we ignore it. 103
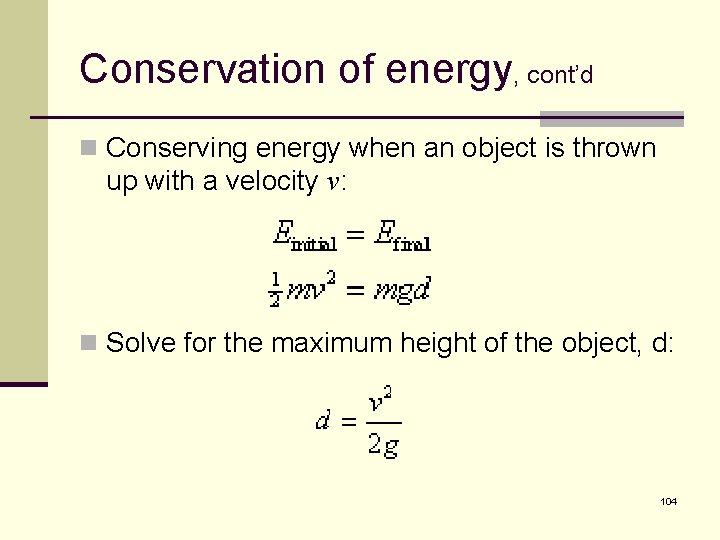
Conservation of energy, cont’d n Conserving energy when an object is thrown up with a velocity v: n Solve for the maximum height of the object, d: 104
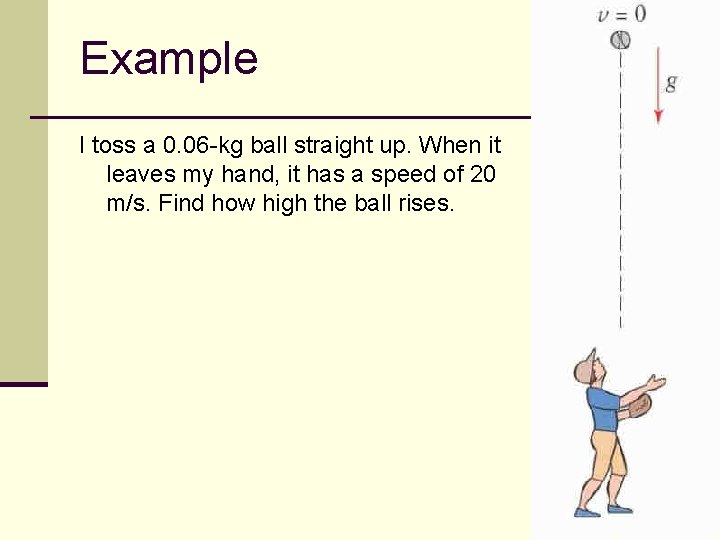
Example I toss a 0. 06 -kg ball straight up. When it leaves my hand, it has a speed of 20 m/s. Find how high the ball rises. 105
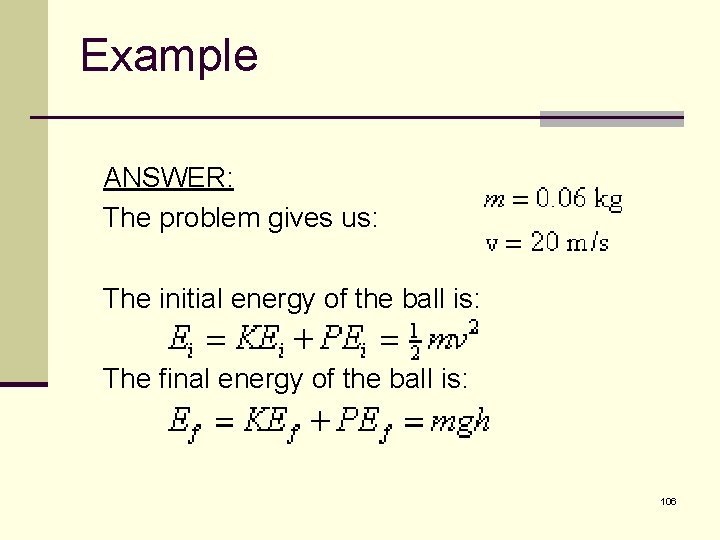
Example ANSWER: The problem gives us: The initial energy of the ball is: The final energy of the ball is: 106
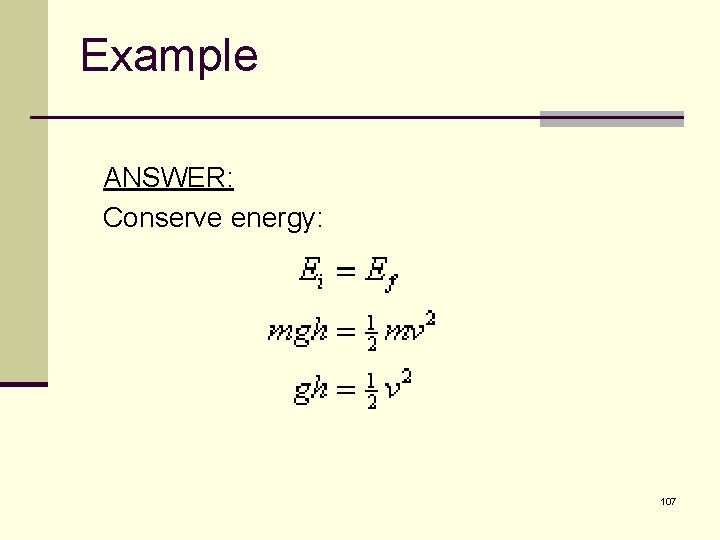
Example ANSWER: Conserve energy: 107
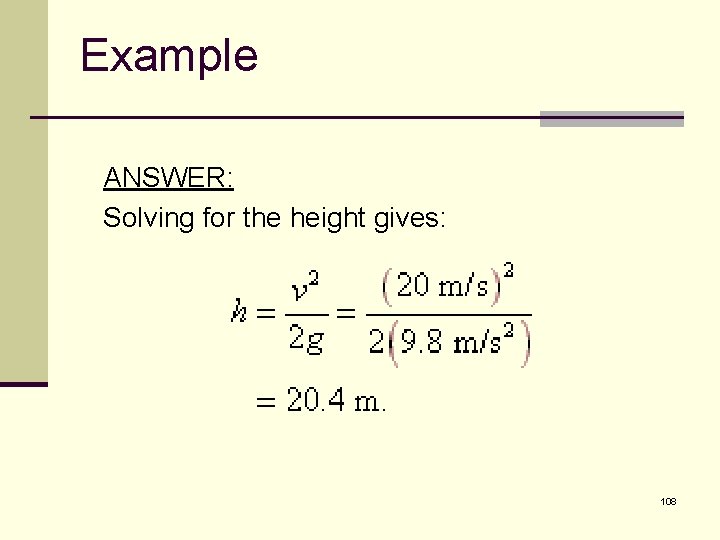
Example ANSWER: Solving for the height gives: 108
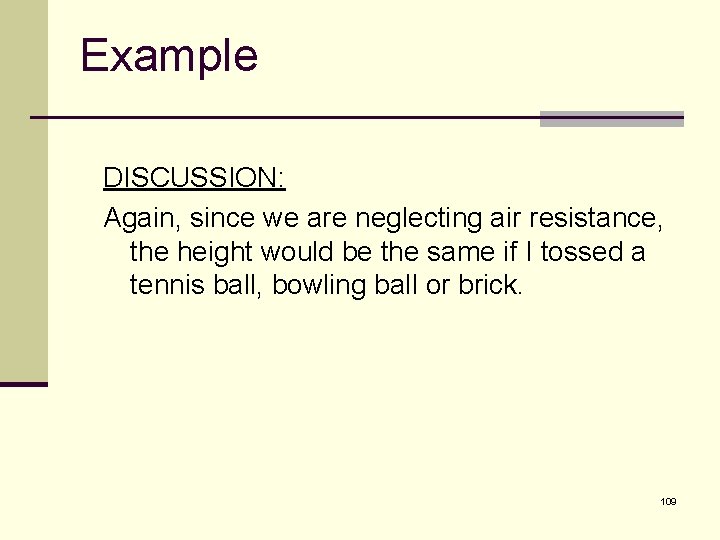
Example DISCUSSION: Again, since we are neglecting air resistance, the height would be the same if I tossed a tennis ball, bowling ball or brick. 109
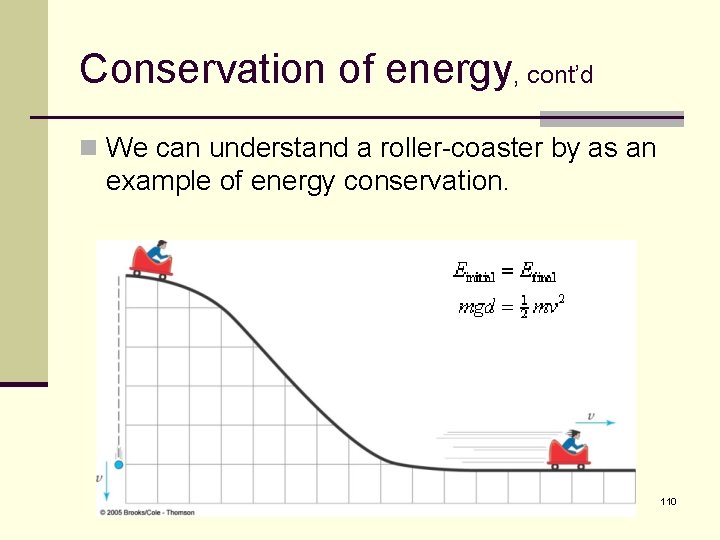
Conservation of energy, cont’d n We can understand a roller-coaster by as an example of energy conservation. 110
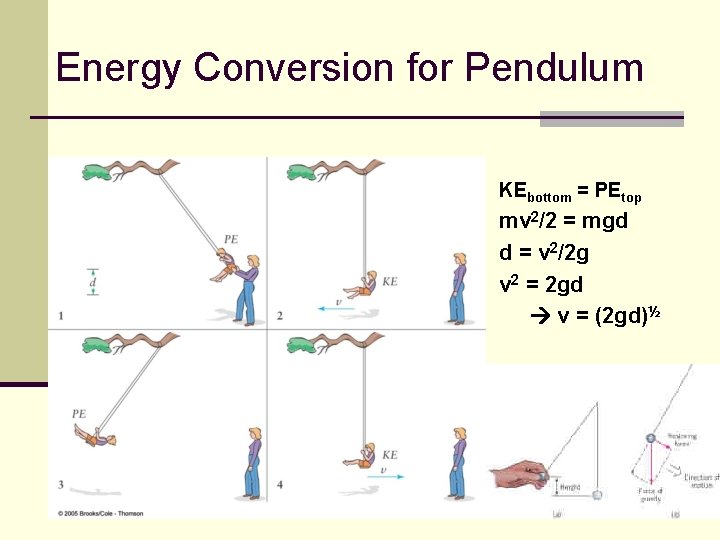
Energy Conversion for Pendulum KEbottom = PEtop mv 2/2 = mgd d = v 2/2 g v 2 = 2 gd v = (2 gd)½ 111
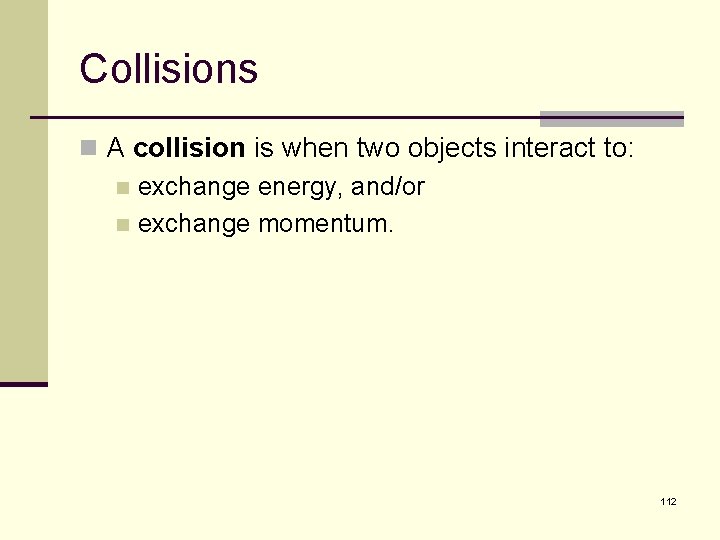
Collisions n A collision is when two objects interact to: n exchange energy, and/or n exchange momentum. 112
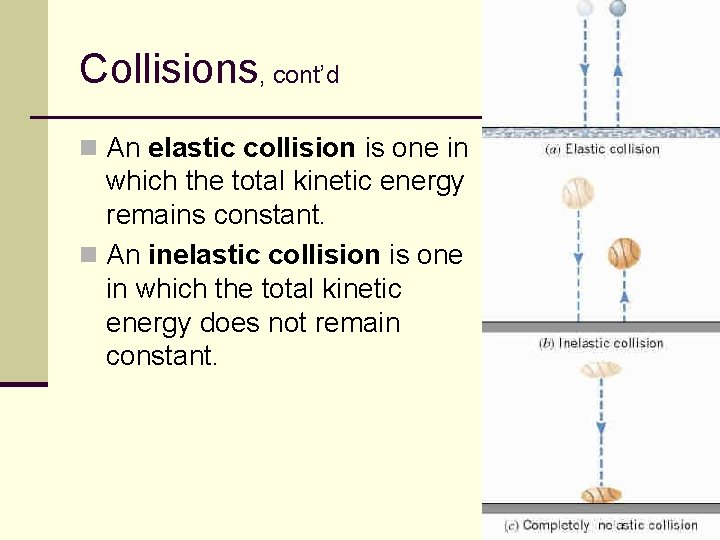
Collisions, cont’d n An elastic collision is one in which the total kinetic energy remains constant. n An inelastic collision is one in which the total kinetic energy does not remain constant. 113
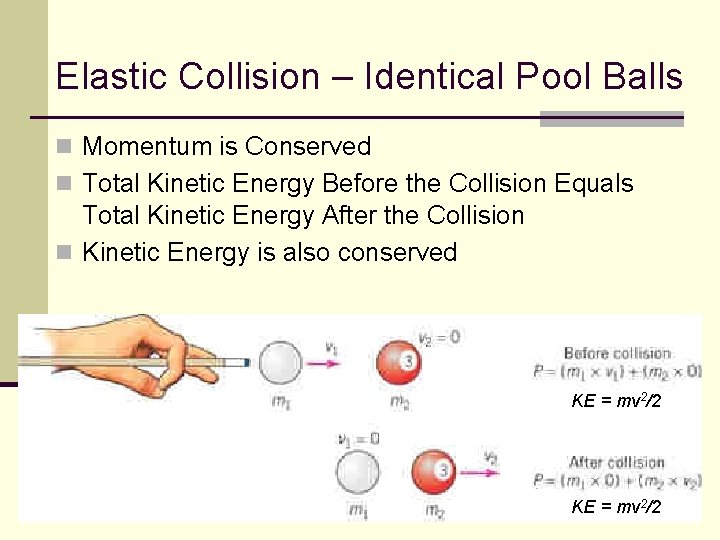
Elastic Collision – Identical Pool Balls n Momentum is Conserved n Total Kinetic Energy Before the Collision Equals Total Kinetic Energy After the Collision n Kinetic Energy is also conserved KE = mv 2/2114
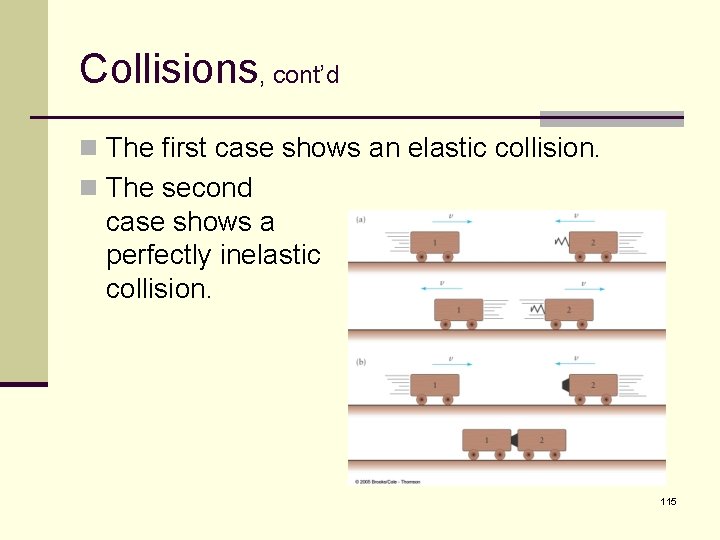
Collisions, cont’d n The first case shows an elastic collision. n The second case shows a perfectly inelastic collision. 115
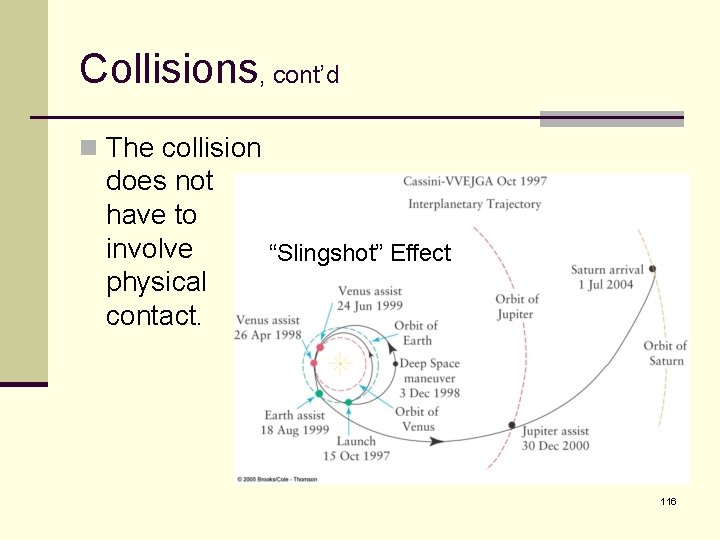
Collisions, cont’d n The collision does not have to involve physical contact. “Slingshot” Effect 116
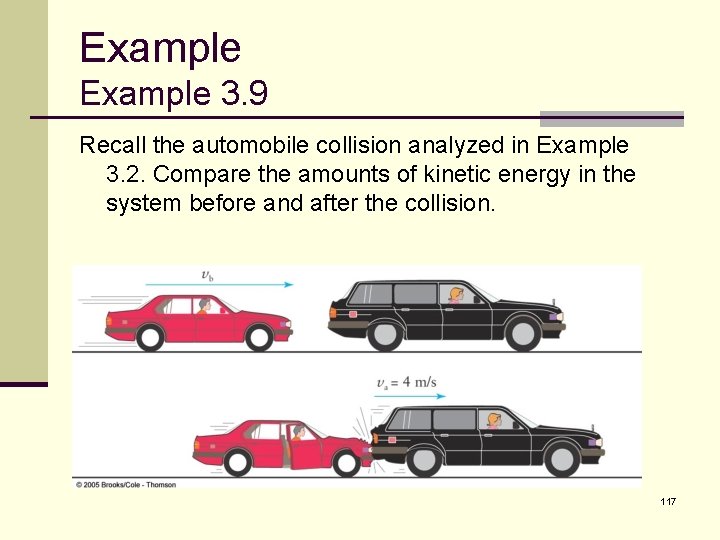
Example 3. 9 Recall the automobile collision analyzed in Example 3. 2. Compare the amounts of kinetic energy in the system before and after the collision. 117
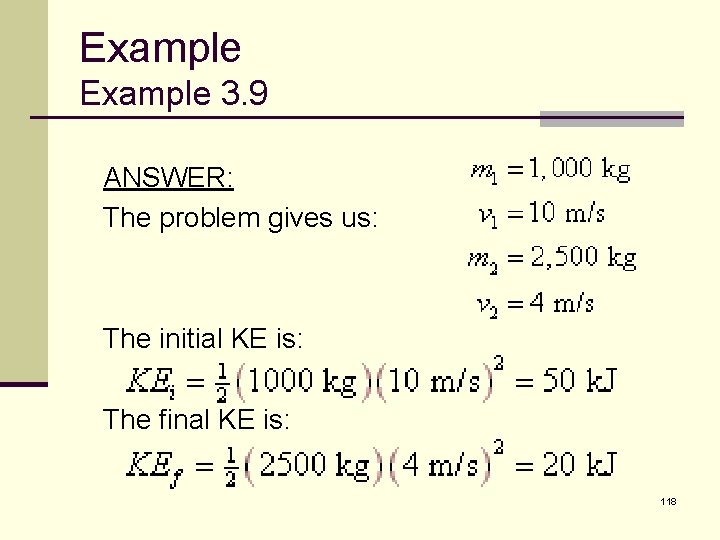
Example 3. 9 ANSWER: The problem gives us: The initial KE is: The final KE is: 118
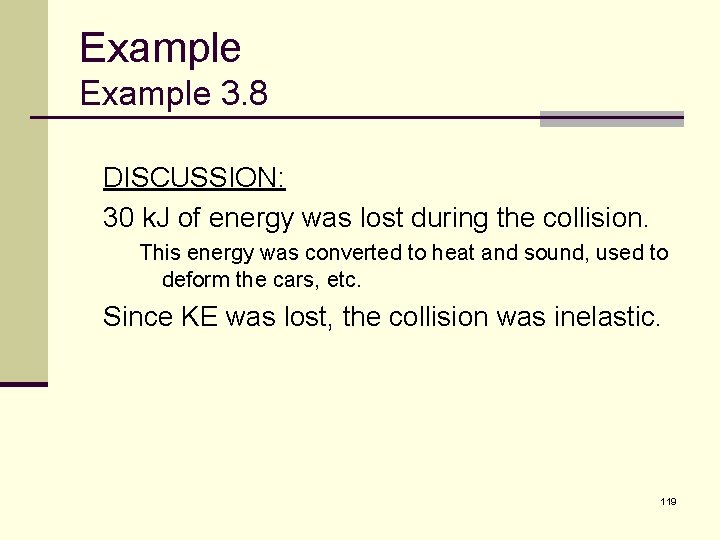
Example 3. 8 DISCUSSION: 30 k. J of energy was lost during the collision. This energy was converted to heat and sound, used to deform the cars, etc. Since KE was lost, the collision was inelastic. 119
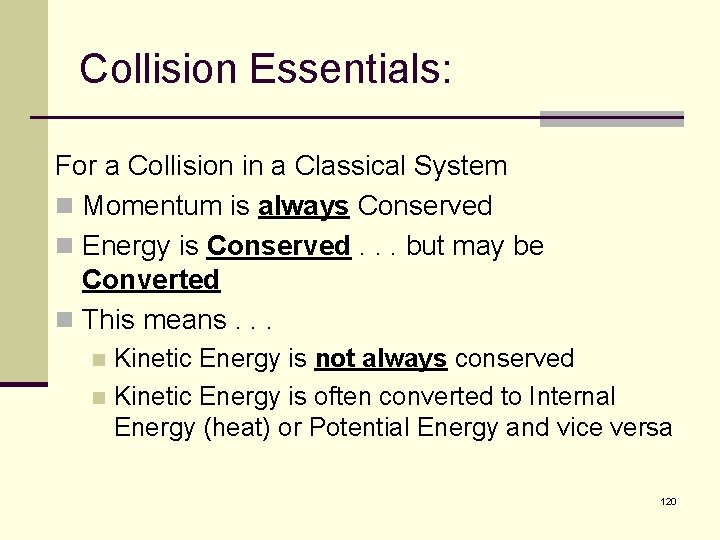
Collision Essentials: For a Collision in a Classical System n Momentum is always Conserved n Energy is Conserved. . . but may be Converted n This means. . . Kinetic Energy is not always conserved n Kinetic Energy is often converted to Internal Energy (heat) or Potential Energy and vice versa n 120
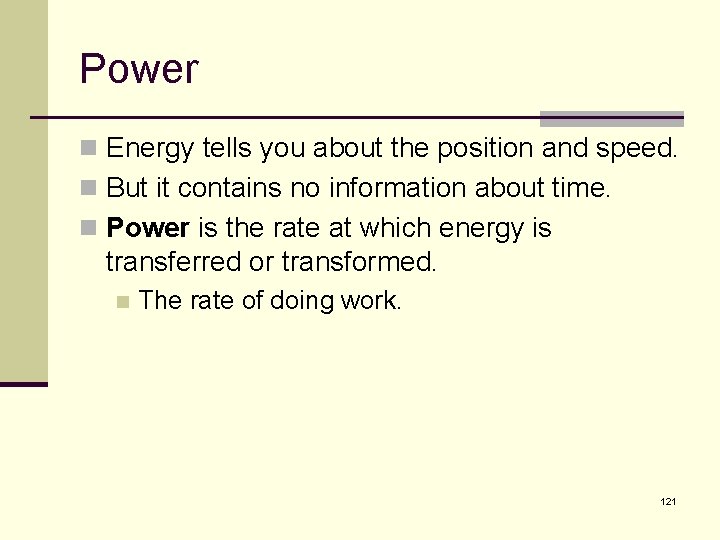
Power n Energy tells you about the position and speed. n But it contains no information about time. n Power is the rate at which energy is transferred or transformed. n The rate of doing work. 121
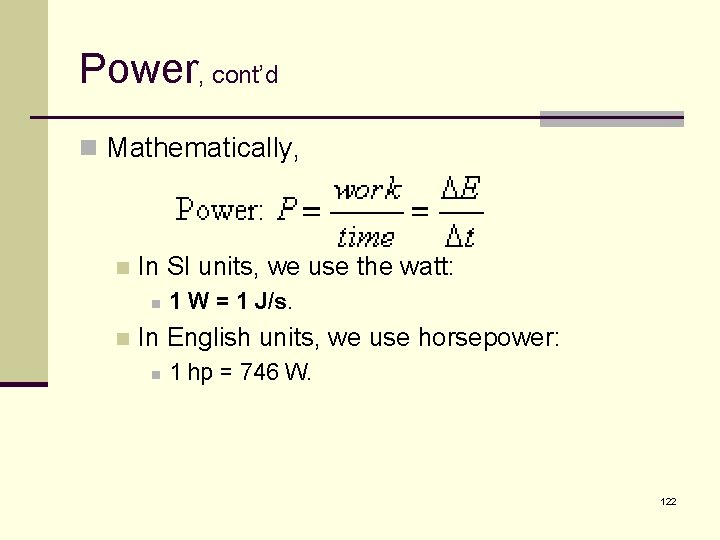
Power, cont’d n Mathematically, n In SI units, we use the watt: n n 1 W = 1 J/s. In English units, we use horsepower: n 1 hp = 746 W. 122
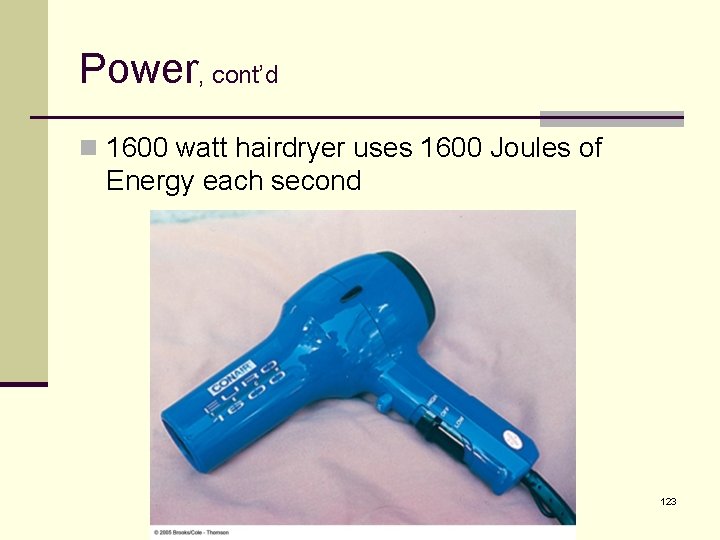
Power, cont’d n 1600 watt hairdryer uses 1600 Joules of Energy each second 123
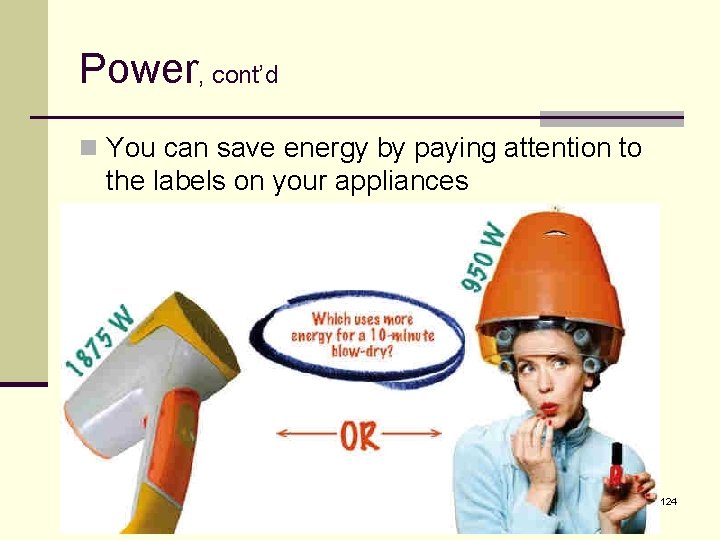
Power, cont’d n You can save energy by paying attention to the labels on your appliances 124
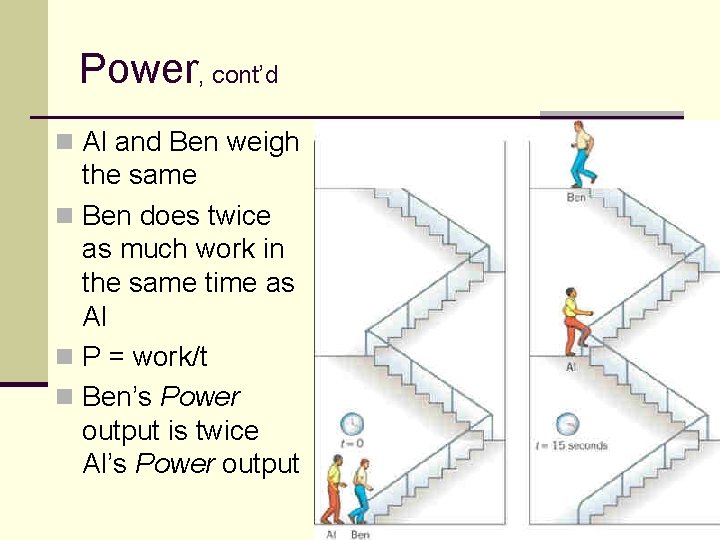
Power, cont’d n Al and Ben weigh the same n Ben does twice as much work in the same time as Al n P = work/t n Ben’s Power output is twice Al’s Power output 125
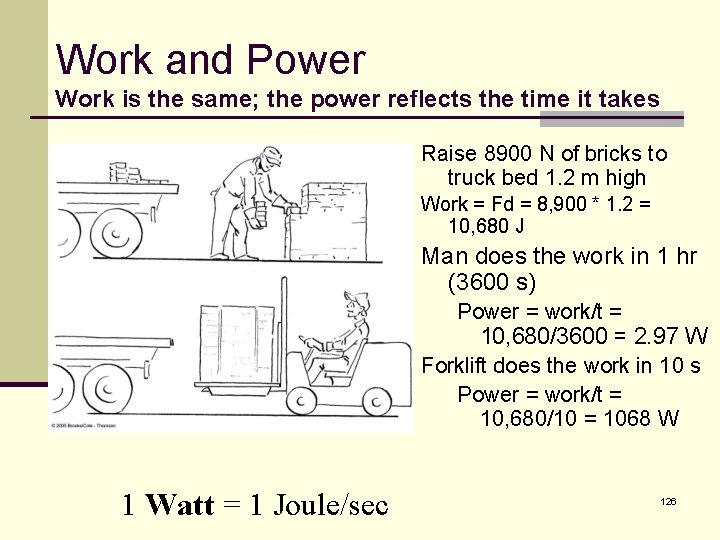
Work and Power Work is the same; the power reflects the time it takes Raise 8900 N of bricks to truck bed 1. 2 m high Work = Fd = 8, 900 * 1. 2 = 10, 680 J Man does the work in 1 hr (3600 s) Power = work/t = 10, 680/3600 = 2. 97 W Forklift does the work in 10 s Power = work/t = 10, 680/10 = 1068 W 1 Watt = 1 Joule/sec 126
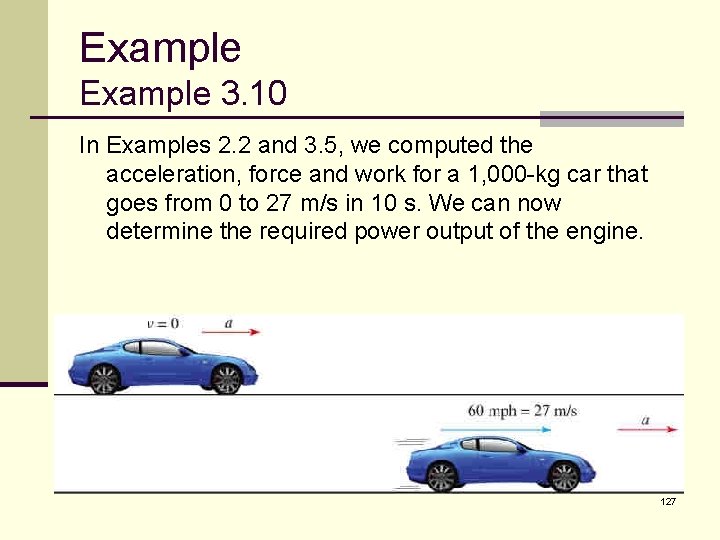
Example 3. 10 In Examples 2. 2 and 3. 5, we computed the acceleration, force and work for a 1, 000 -kg car that goes from 0 to 27 m/s in 10 s. We can now determine the required power output of the engine. 127
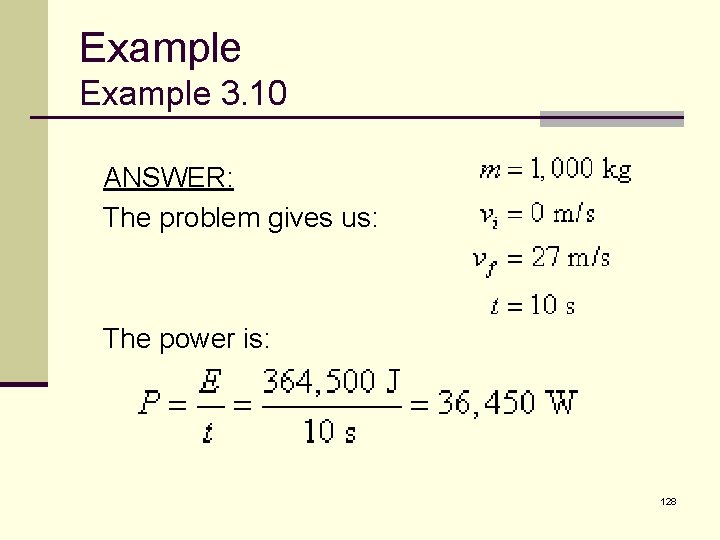
Example 3. 10 ANSWER: The problem gives us: The power is: 128
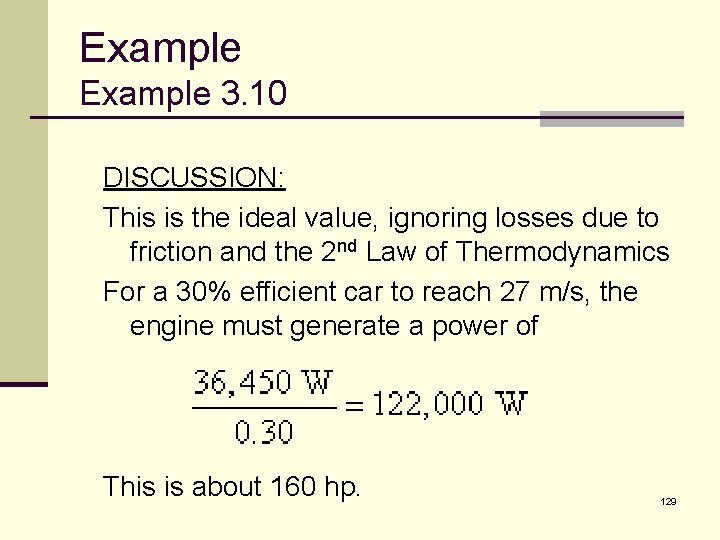
Example 3. 10 DISCUSSION: This is the ideal value, ignoring losses due to friction and the 2 nd Law of Thermodynamics For a 30% efficient car to reach 27 m/s, the engine must generate a power of This is about 160 hp. 129
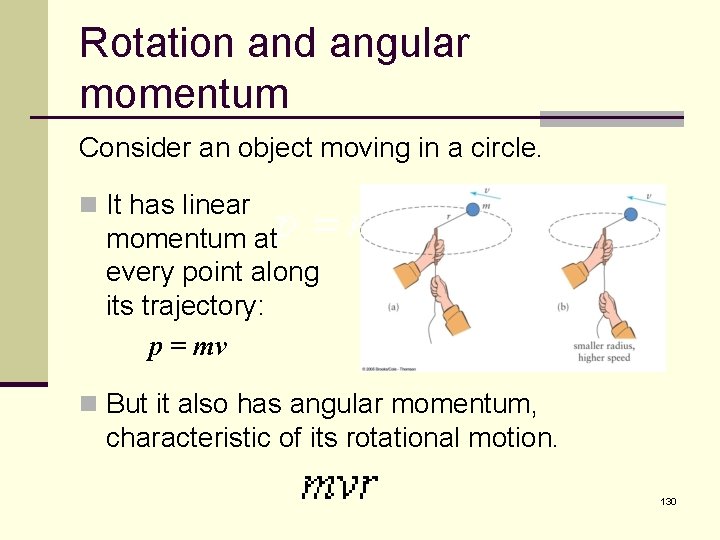
Rotation and angular momentum Consider an object moving in a circle. n It has linear momentum at every point along its trajectory: p = mv n But it also has angular momentum, characteristic of its rotational motion. 130
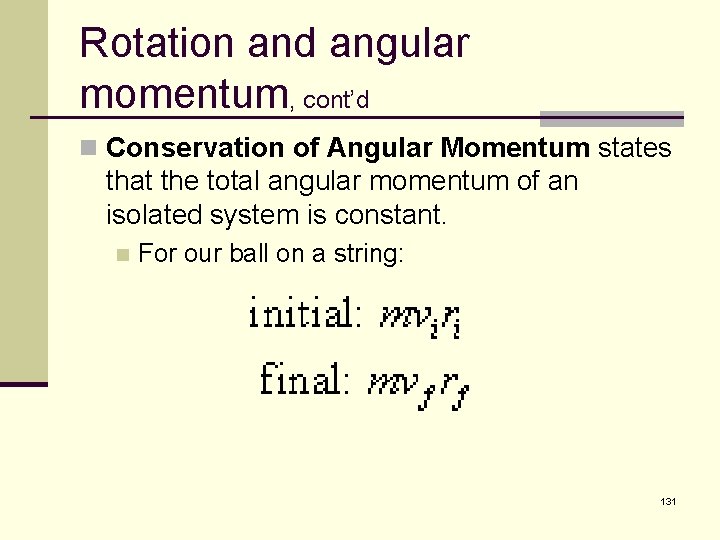
Rotation and angular momentum, cont’d n Conservation of Angular Momentum states that the total angular momentum of an isolated system is constant. n For our ball on a string: 131
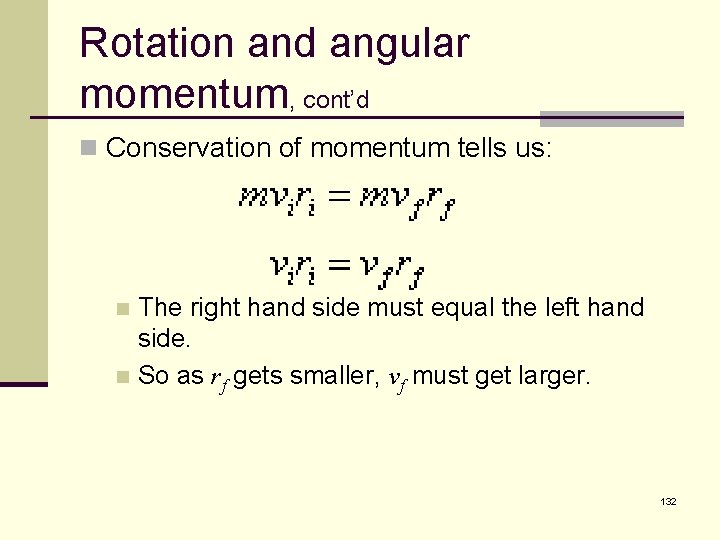
Rotation and angular momentum, cont’d n Conservation of momentum tells us: The right hand side must equal the left hand side. n So as rf gets smaller, vf must get larger. n 132
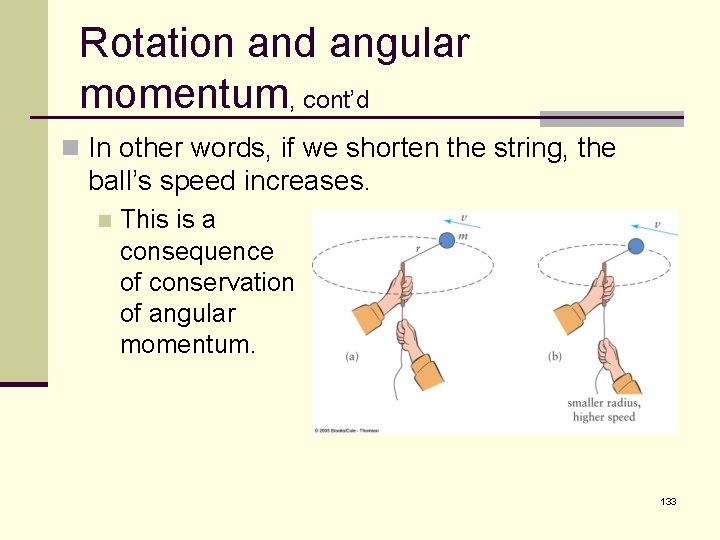
Rotation and angular momentum, cont’d n In other words, if we shorten the string, the ball’s speed increases. n This is a consequence of conservation of angular momentum. 133
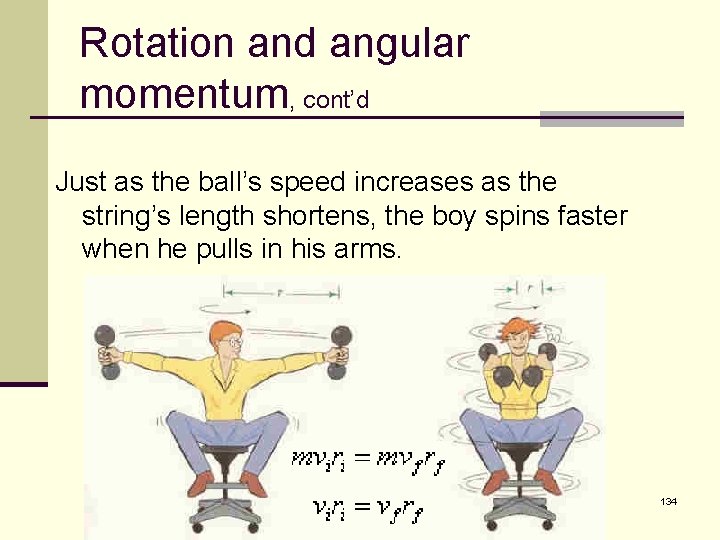
Rotation and angular momentum, cont’d Just as the ball’s speed increases as the string’s length shortens, the boy spins faster when he pulls in his arms. 134
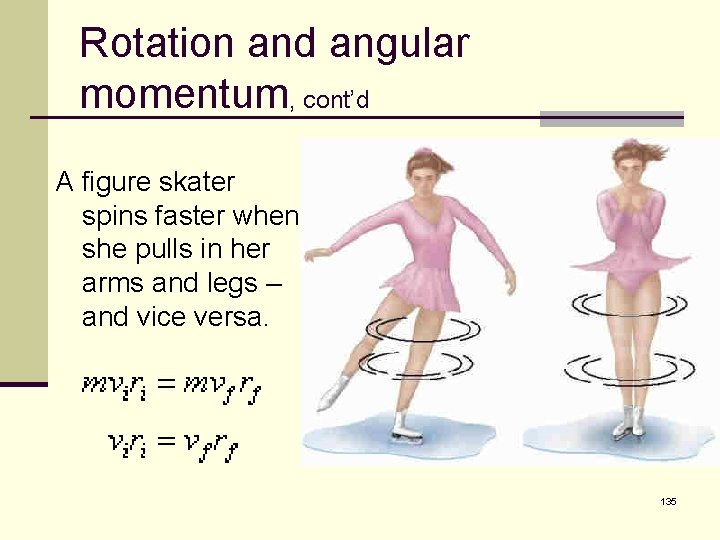
Rotation and angular momentum, cont’d A figure skater spins faster when she pulls in her arms and legs – and vice versa. 135
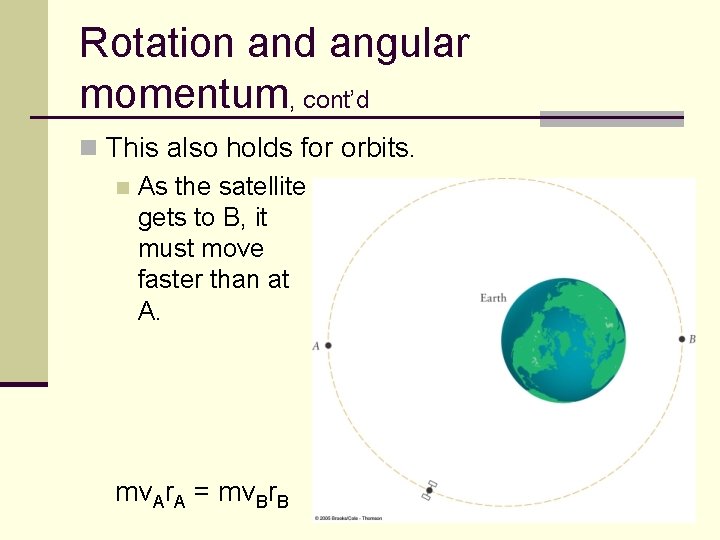
Rotation and angular momentum, cont’d n This also holds for orbits. n As the satellite gets to B, it must move faster than at A. mv. Ar. A = mv. Br. B 136
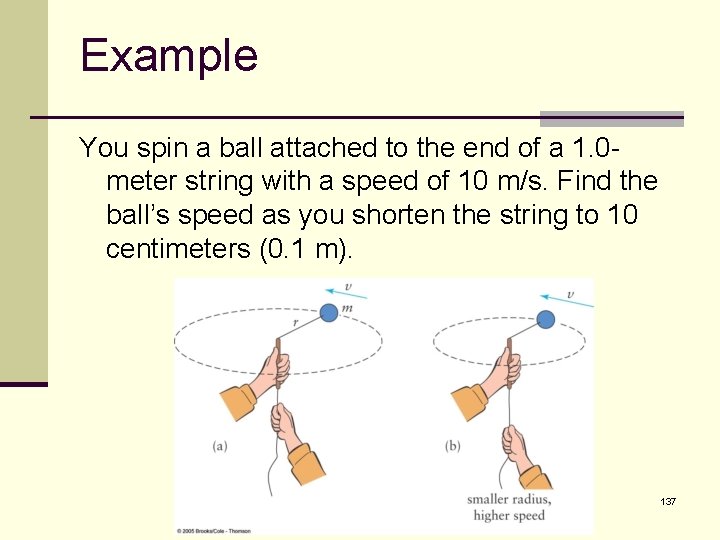
Example You spin a ball attached to the end of a 1. 0 meter string with a speed of 10 m/s. Find the ball’s speed as you shorten the string to 10 centimeters (0. 1 m). 137
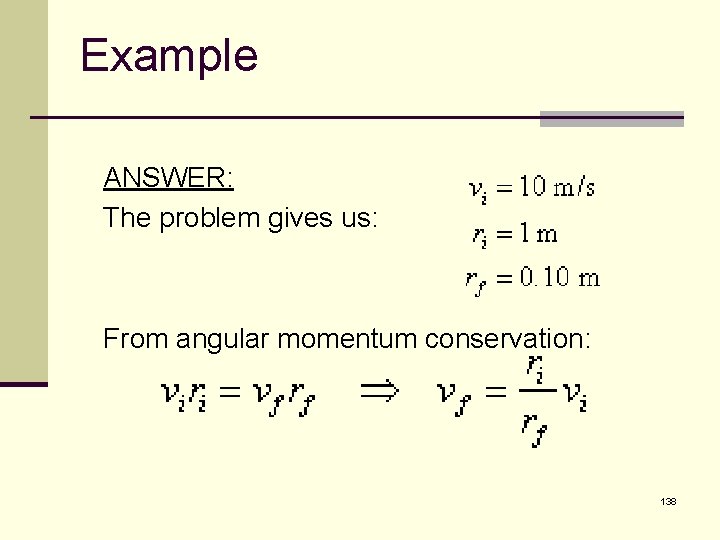
Example ANSWER: The problem gives us: From angular momentum conservation: 138
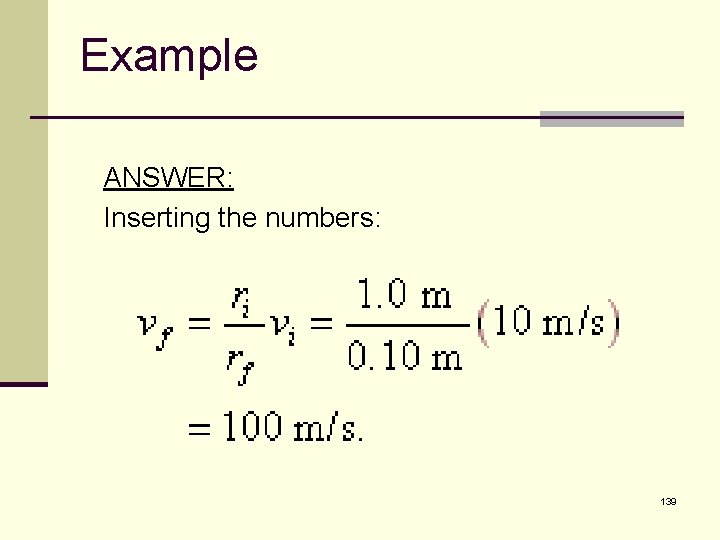
Example ANSWER: Inserting the numbers: 139
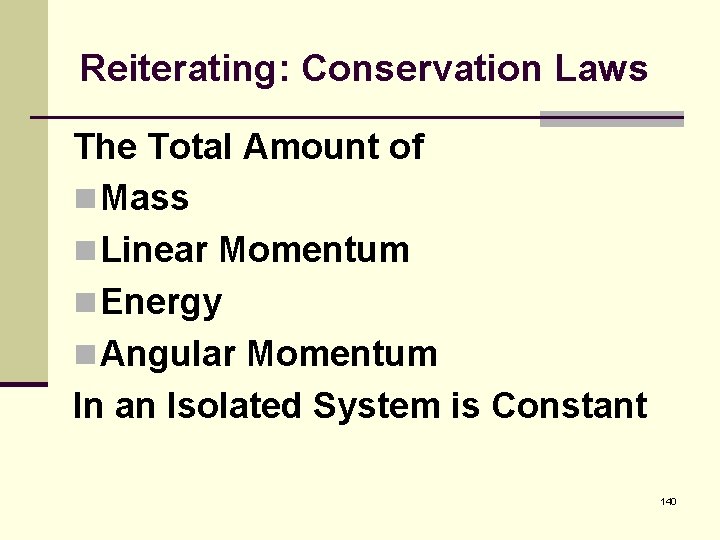
Reiterating: Conservation Laws The Total Amount of n Mass n Linear Momentum n Energy n Angular Momentum In an Isolated System is Constant 140
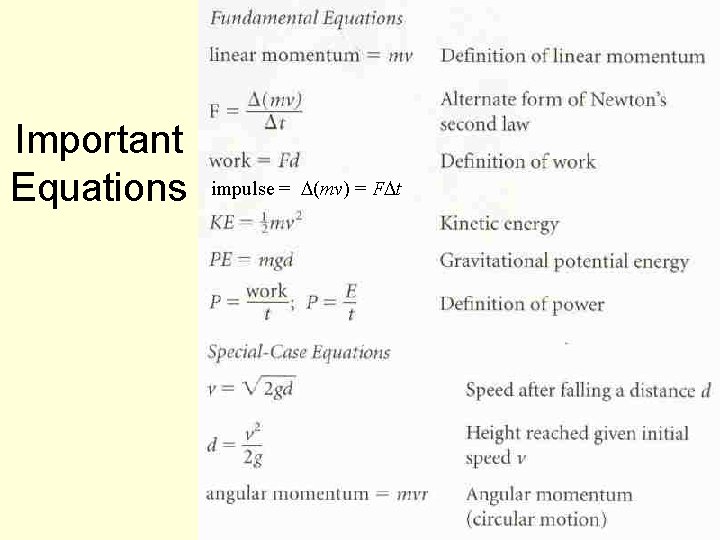
Important Equations impulse = D(mv) = FDt 141
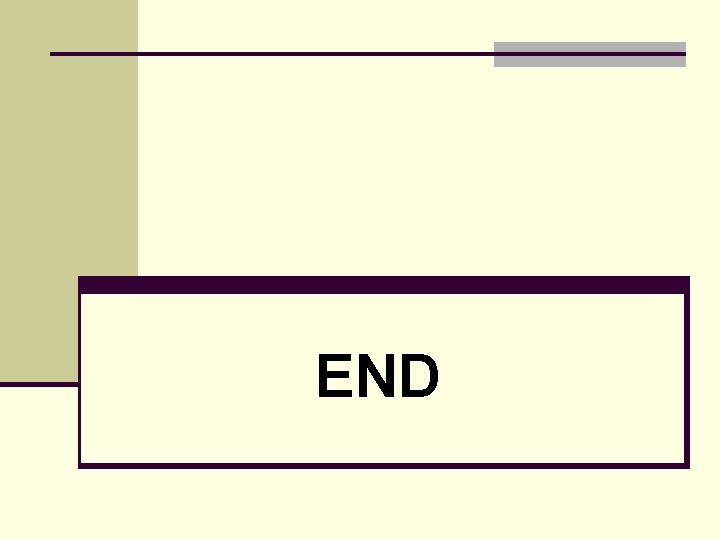
END
Phy 110
Introduction to physics 110
Numero niss
110-000-110 & 111-000-111
Phy 131 past papers
Pa msu
Phy theorem
Rotational statics
Phy theorem
Phy 113 past questions and answers
Phy 121 asu
Ddr phy architecture
Phy 205
Site:slidetodoc.com
Phy 2049
Phy
Phy
Phy
Atm basics
Fizik ii
Phy 2049
Phy 1214
Phy 1214
Phy
Phy
Phy
Life phy
Phy1501
Phy 1214
2012 phy
Phy-105 5 discussion
Loncapa fsu
Phy 212
General physics chapters
Vx=vox+axt
Nearsightedness physics
Phy 132
Phy 1214
Phy108
Phy tgen
Why does it happen
University physics with modern physics fifteenth edition
Physics research questions ib
Introduction to static equilibrium mastering physics
Modern physics introduction
Modern physics introduction
Modern physics introduction
Device physics
Scanything
Physics chapter 1 introduction and mathematical concepts
An introduction to atmospheric physics
Kinematics ppt free download
Troop 110
Silinder logam (t1 = 250°c) dengan diameter 10 cm
Nn omb
Toxicology effects
Miller indices 111
What is linear density
Uma onda possui uma frequencia angular de 110 rad/s
Hızınız 110 yavaş aq
Eecs 110
Cit 110
Cis 110 upenn
Hexadecimal number
Kısmi basınç formül
Fahrenheit 451 pages 93-110 summary
110 decibels
Psalm 110 1
Transversal of parallel lines find angle measures
If r s t u v 110
Preterite of regular verbs (p. 110)
Ultibro breezhaler 110/50
It 110
Inf 110
Salmo 110 1
Csce 110 tamu syllabus
Tehilim 110
Cis 110 syllabus
Csct-110
Iec tc110
Raoult's law and dalton's law
011 101 110
Nec 110-26
Priesthood keys restored in kirtland temple
Peraturan-peraturan elektrik 1994
Doctrine and covenants section 110
Head tilt chin lift jaw thrust
111 family of directions
Nst 110
Dpt 110
Dpt 110
Linear atomic density
Ios 110
Ellos _________ al golf.
110 ideas
Cpit110
100 101 102
Berapa kapasitas kalor dari 5 kg
Wda110wcs
110 baud
Hkl-01
Konversi bilangan biner ke oktal dari 1101112 adalah
El señor recuerda siempre su alianza
Explain the following lines (104-110):
192-168-1-20
Bisp 195 ucsd
Ios 110
Afji 48-110
If , what is ? 20° 70° 110° 130°
110
How to find one mole of a compound
Floating gate transistor animation
Lmt 121
Ece 211
Cpit 110
Cpit 110
Mcb 110
Cmu 15-110
Speech 110
Eosc 111 ubc
Lesson 110
Cbt 110
Requirements management training
Regular verbs in the preterite
Bcc 110 111 slip system
Definite article spanish
Kin 110
Kin 110
Mind map gerak harmonik sederhana
Bio 110 psu
Materi garis dan sudut kelas 7
Cps 310
Visa 110
Dot/faa/ar-02/109
Sara yousef
110 wp pv module
Csis 110
Csce 110 tamu
Csc 110 university of arizona
Cps 110
Cpit 110
Cpit 110
Afosh std 48-20