CHM 5681 Timeresolved emission Source Clock hn Sample
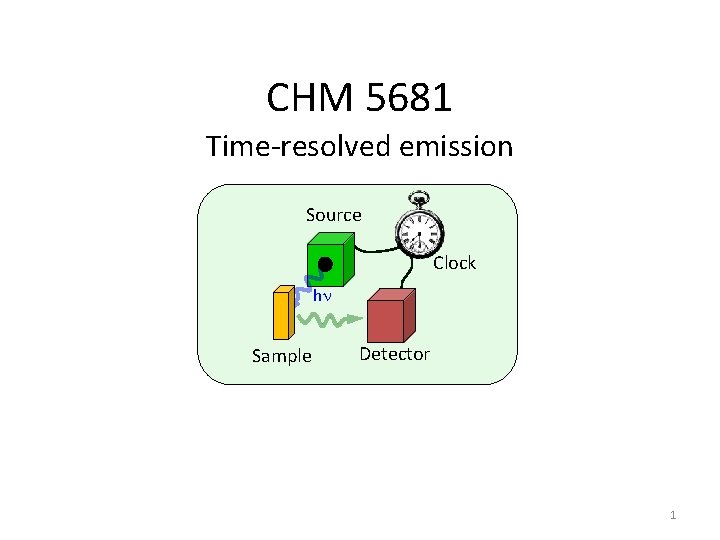
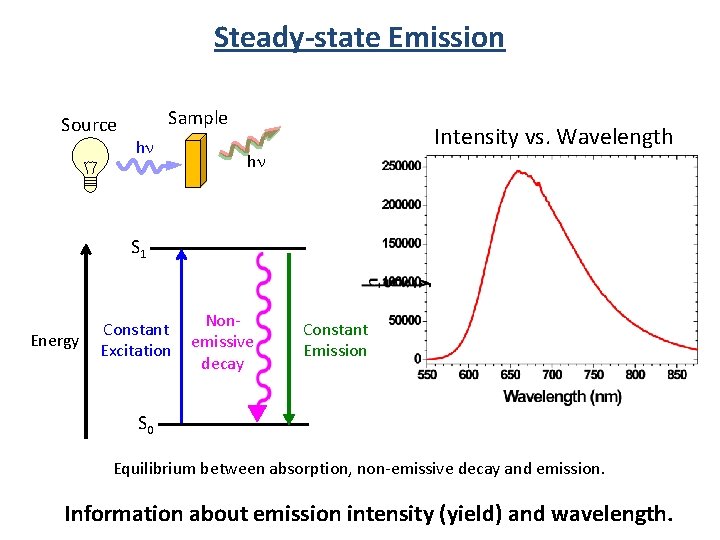
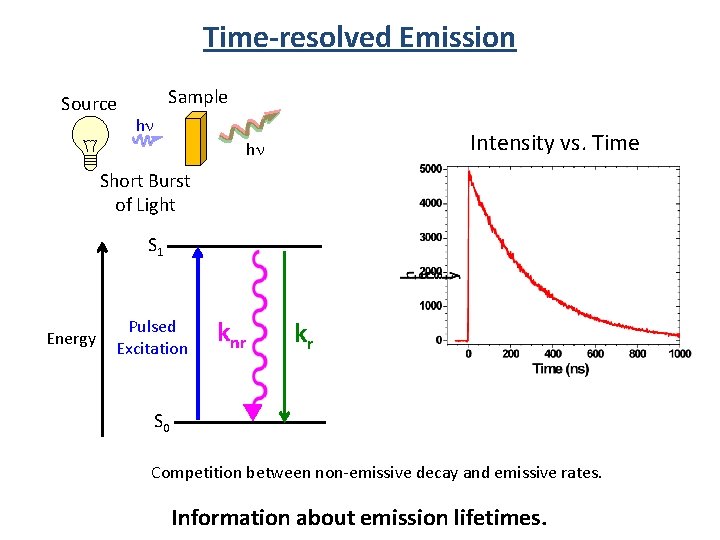
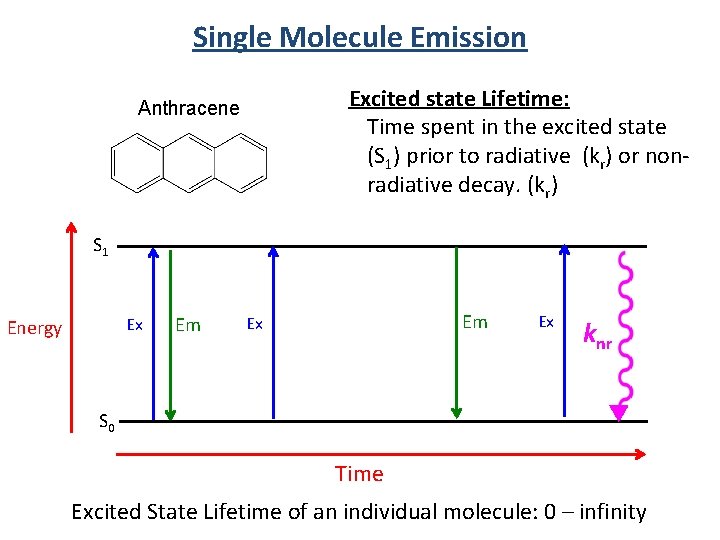
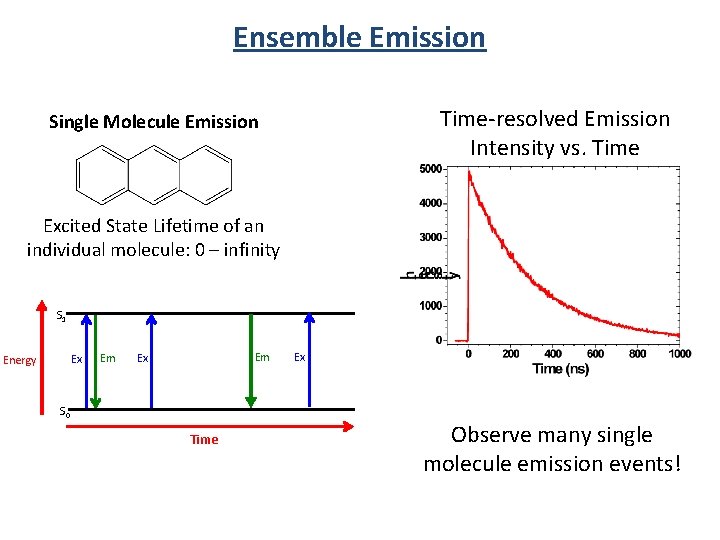
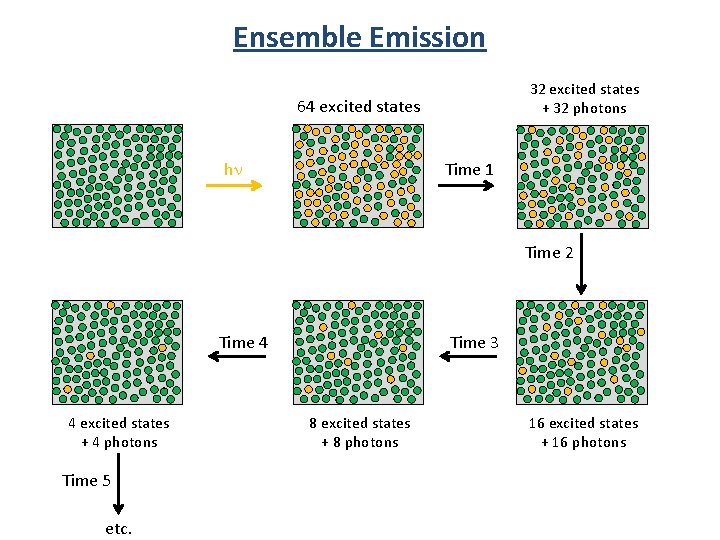
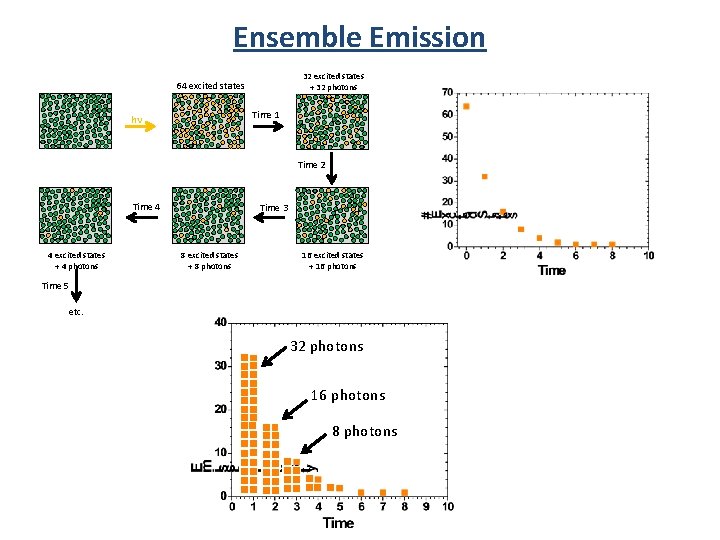
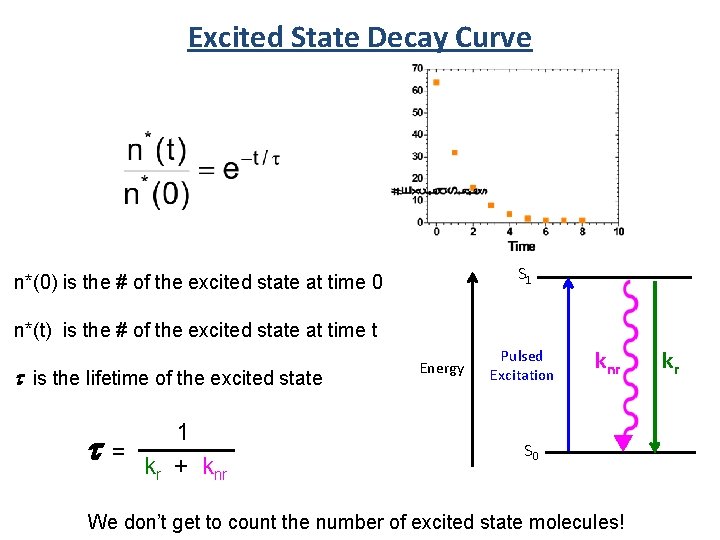
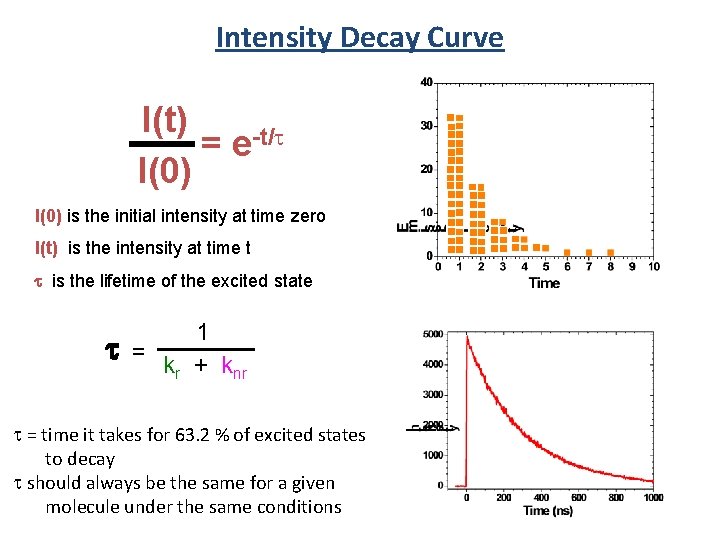
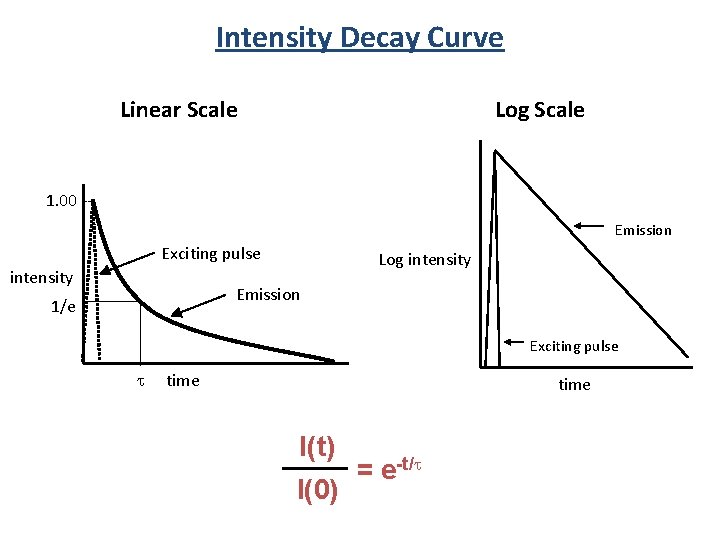
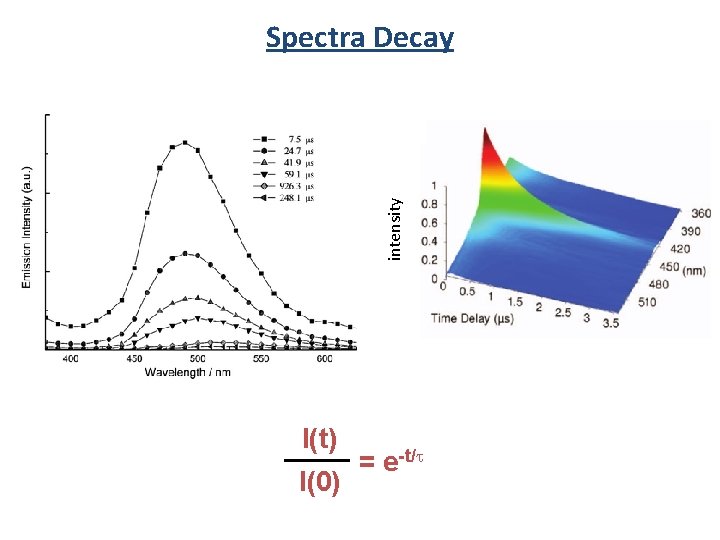
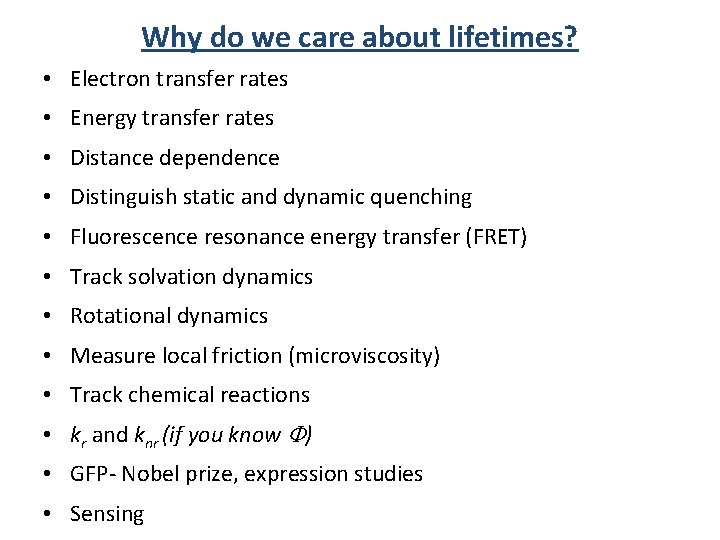
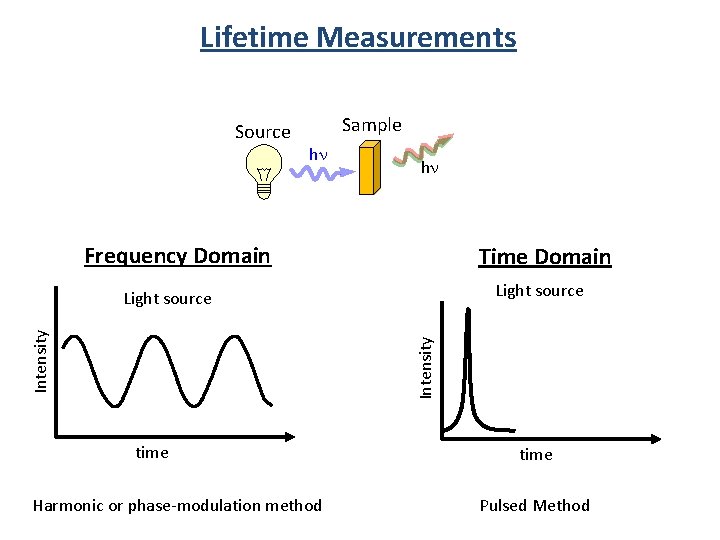
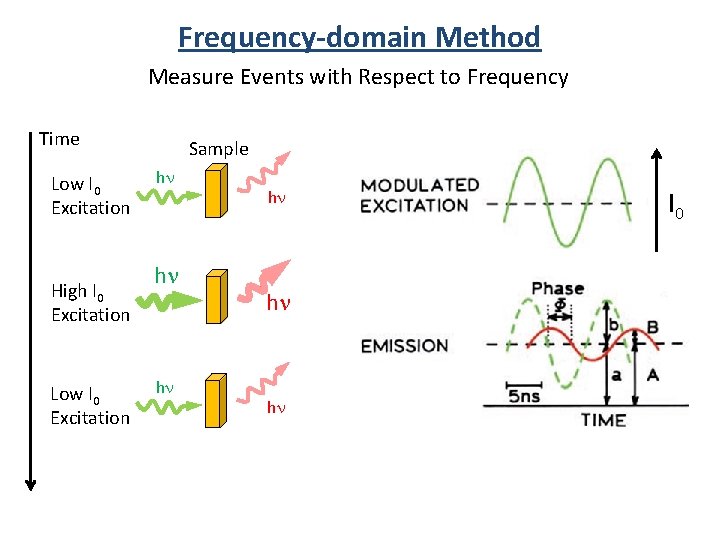
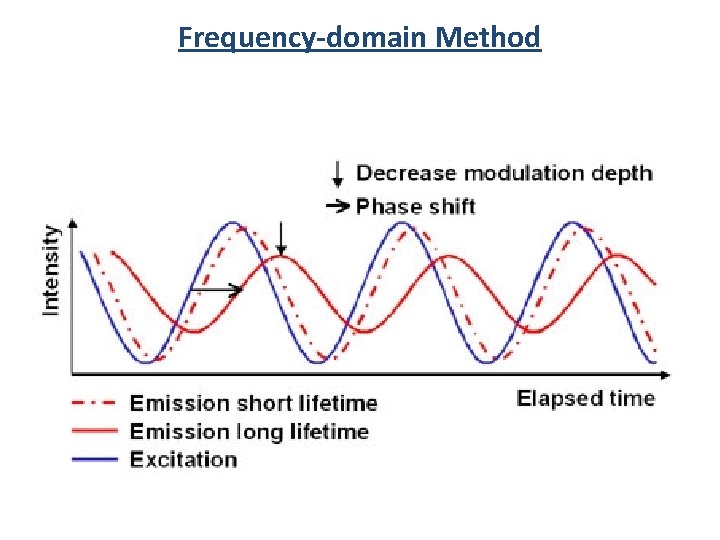
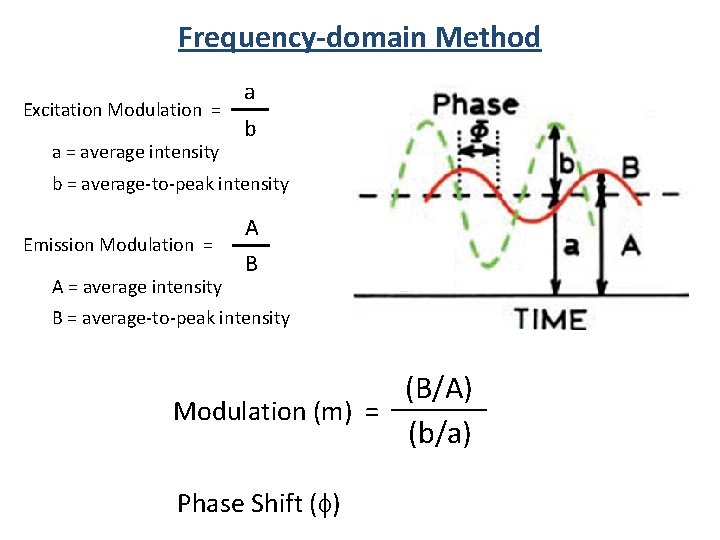
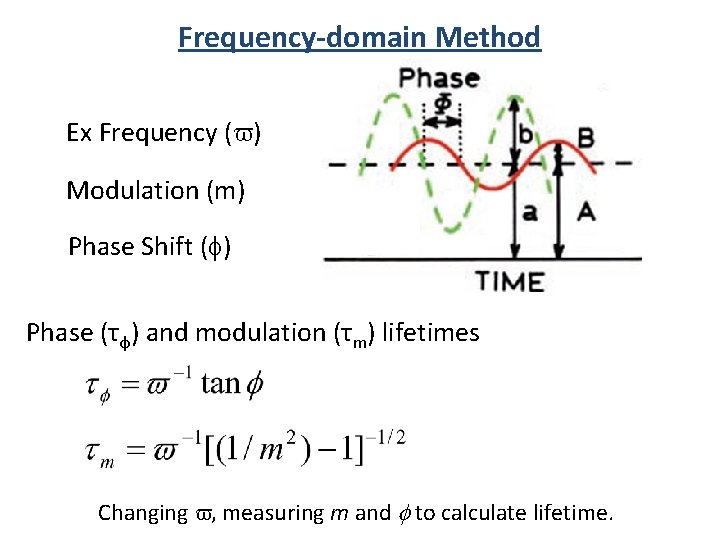
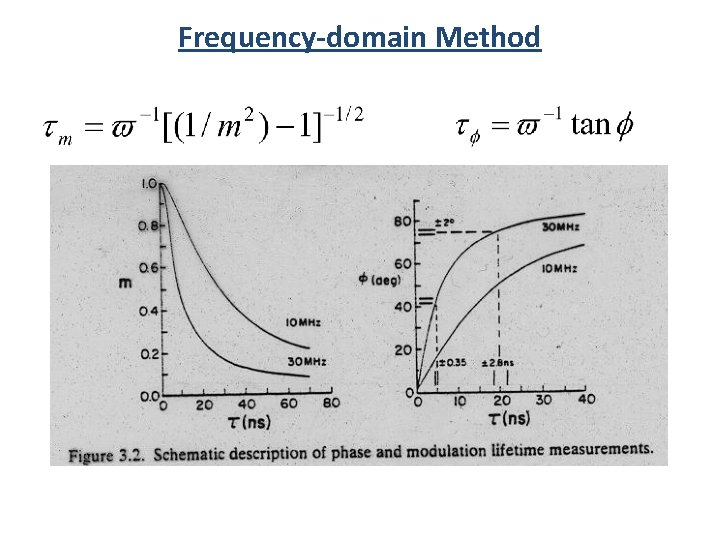
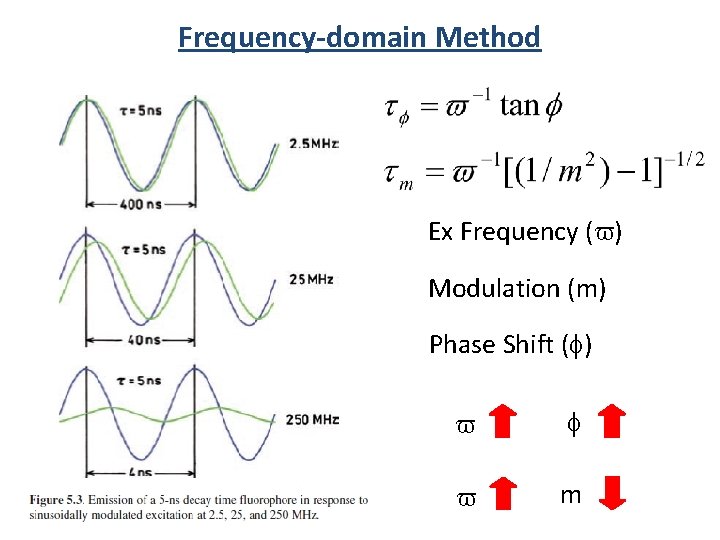
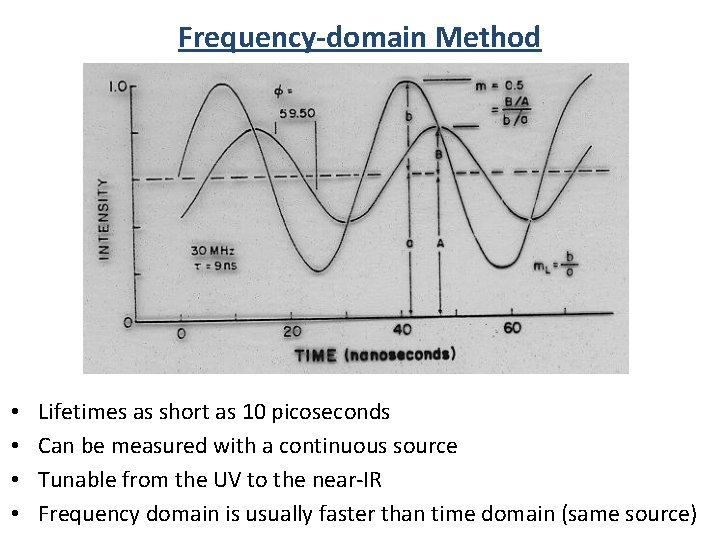
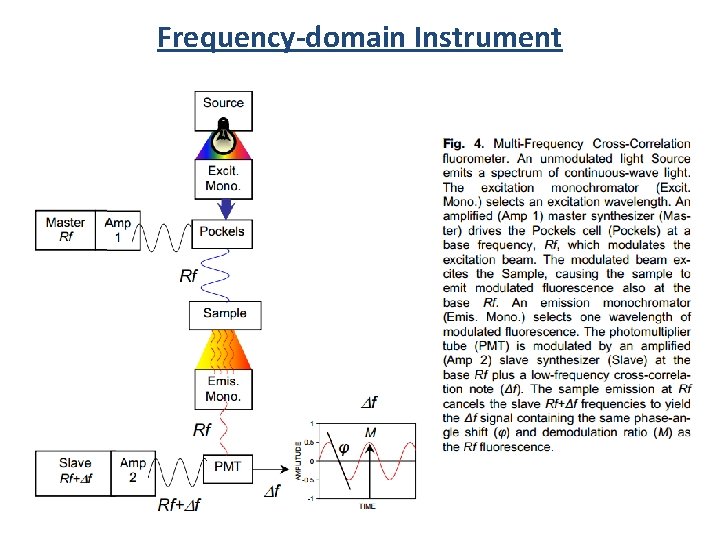
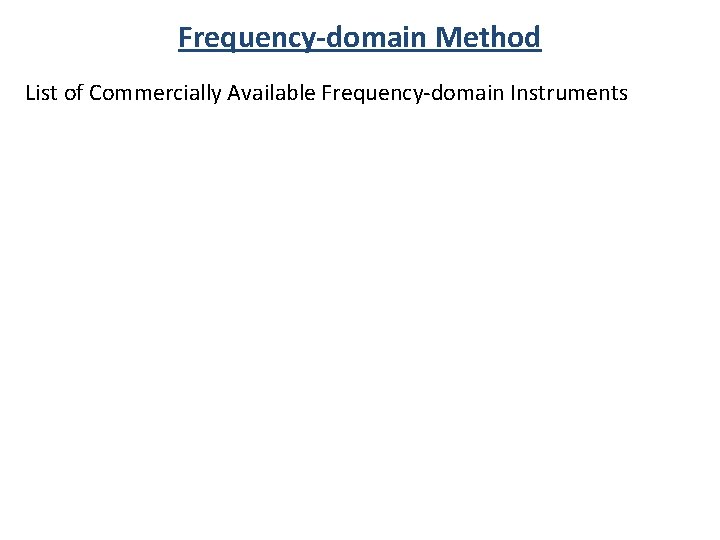
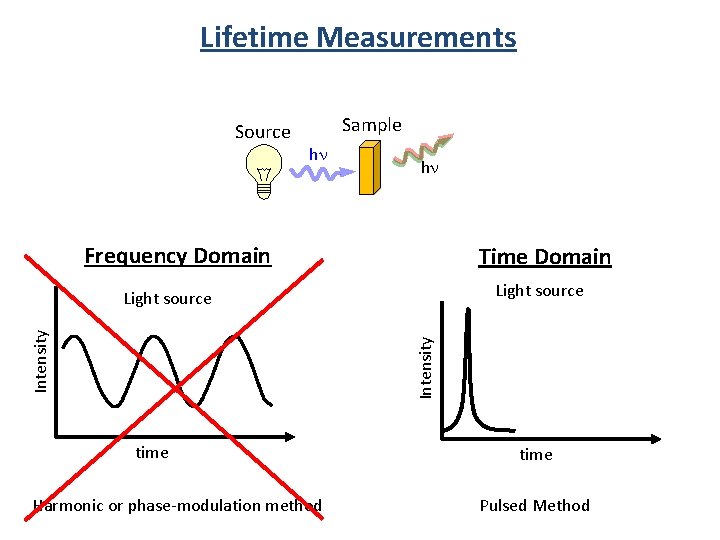
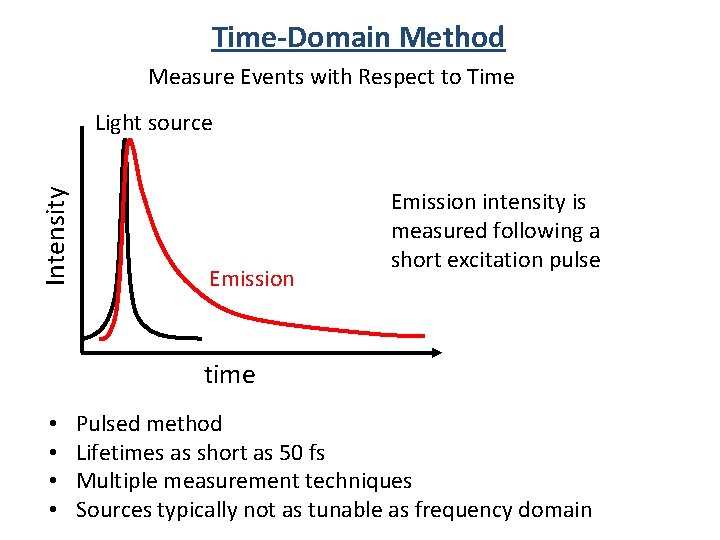
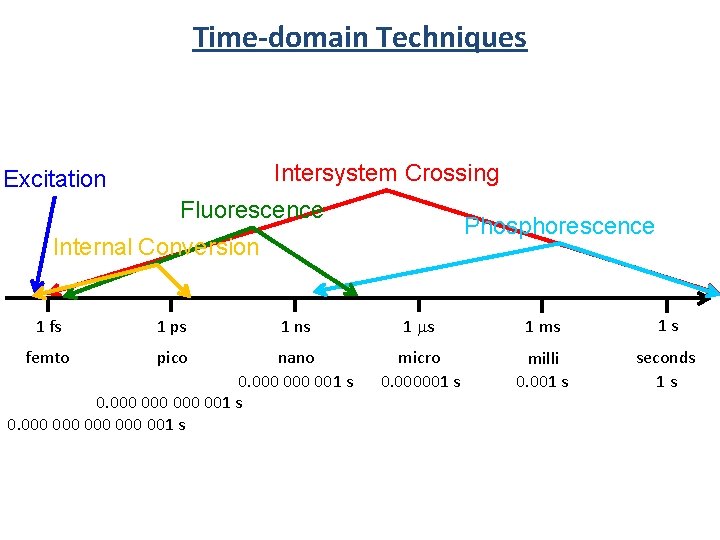
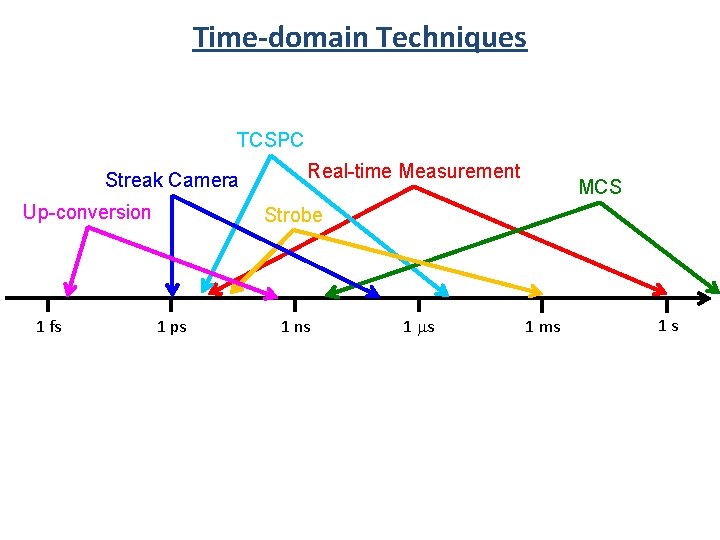
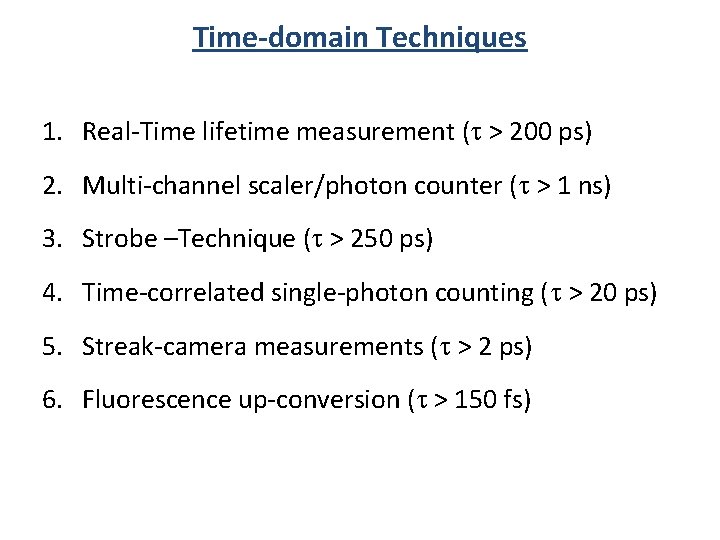
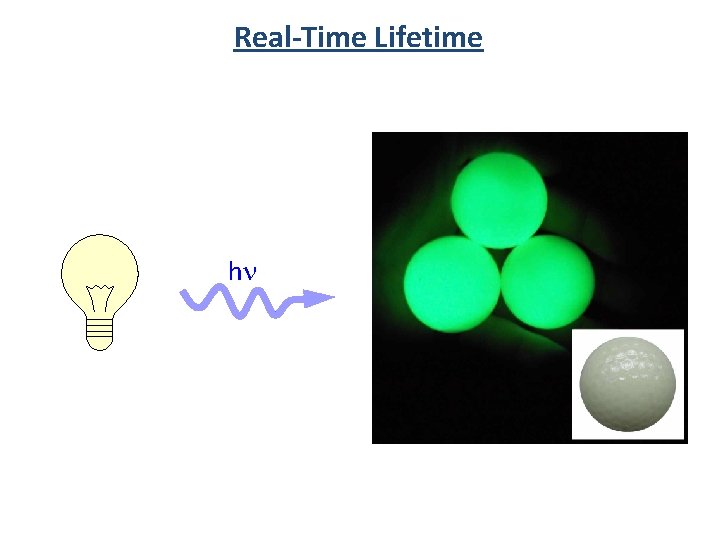
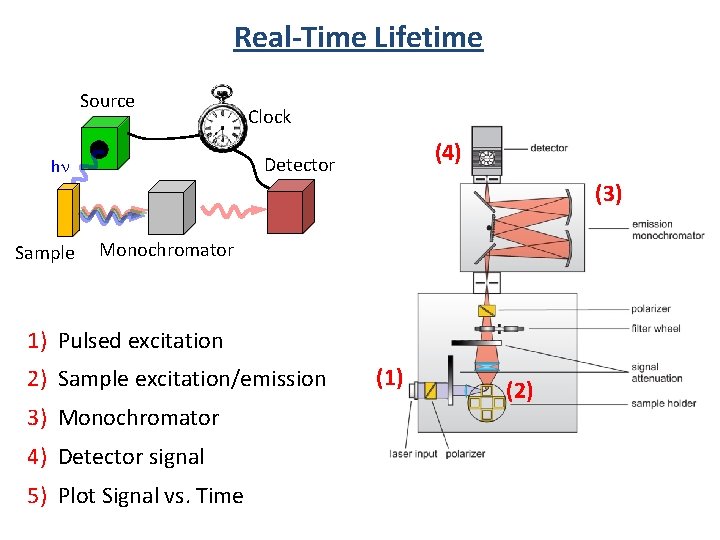
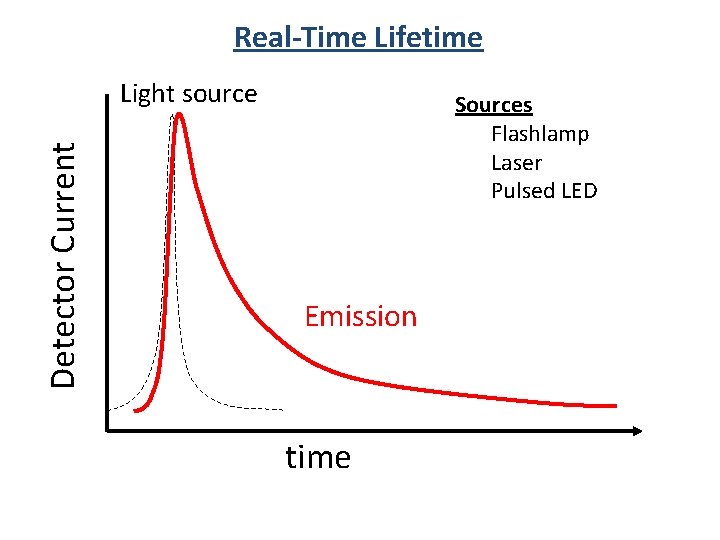
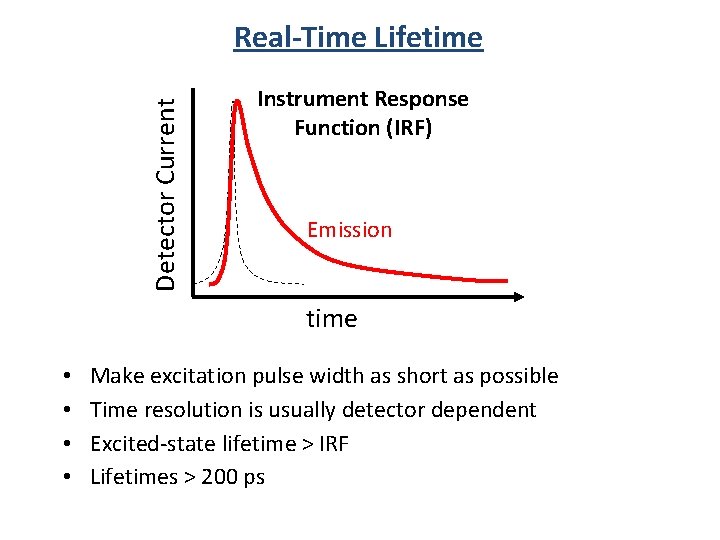
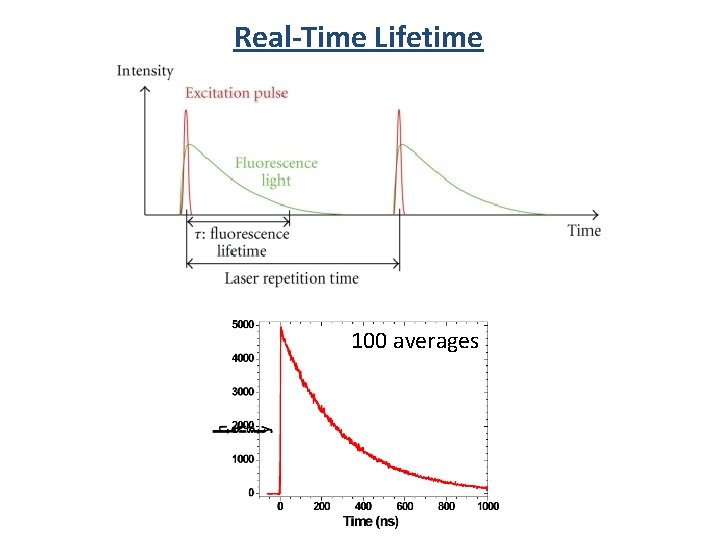
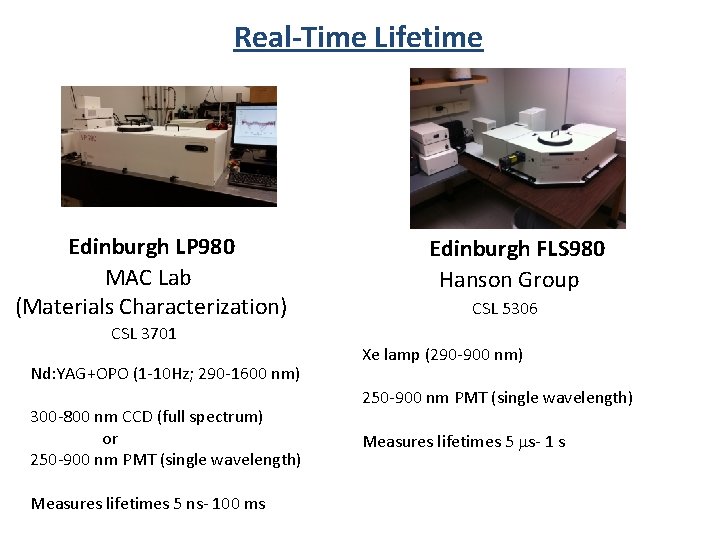
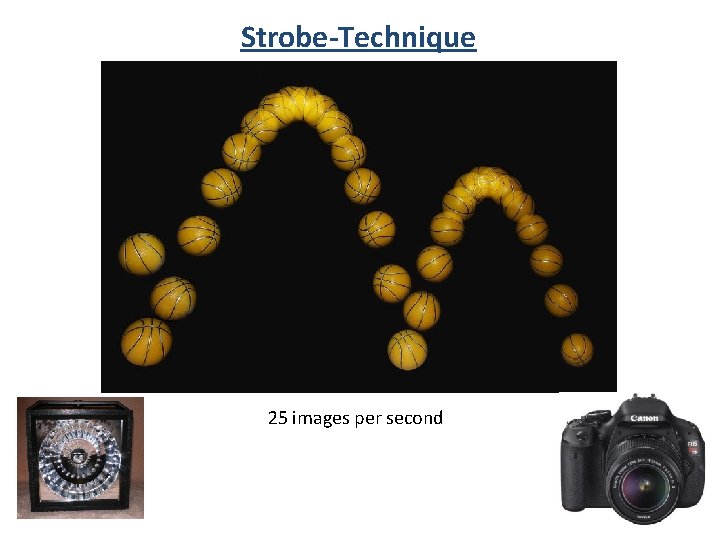
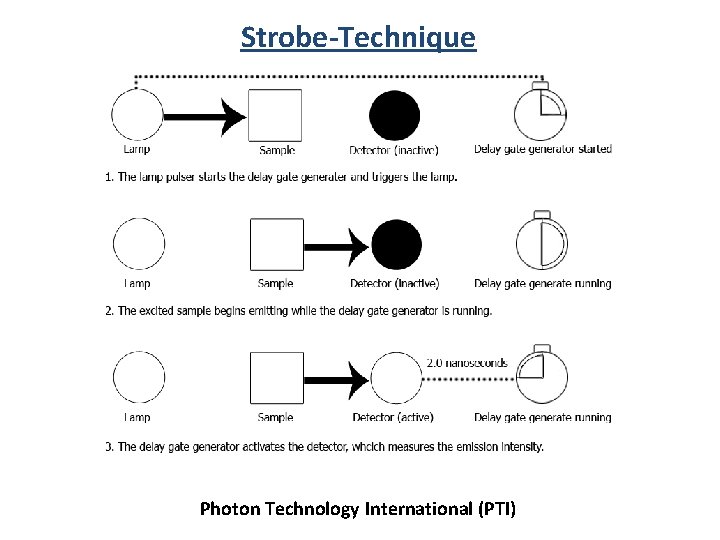
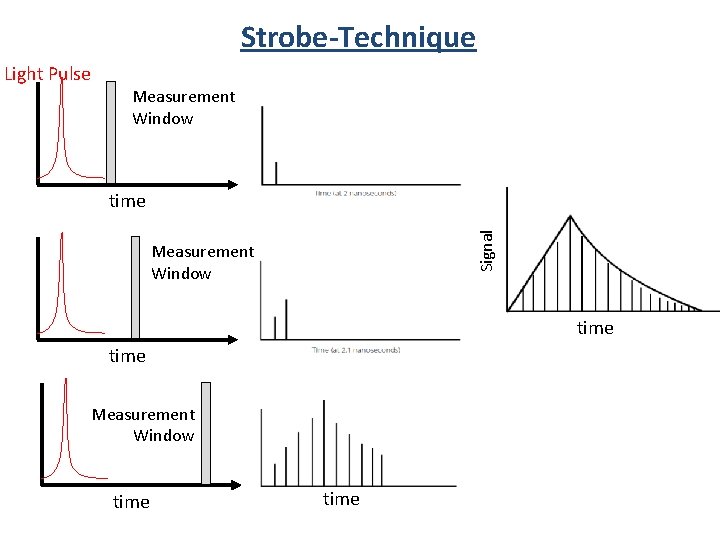
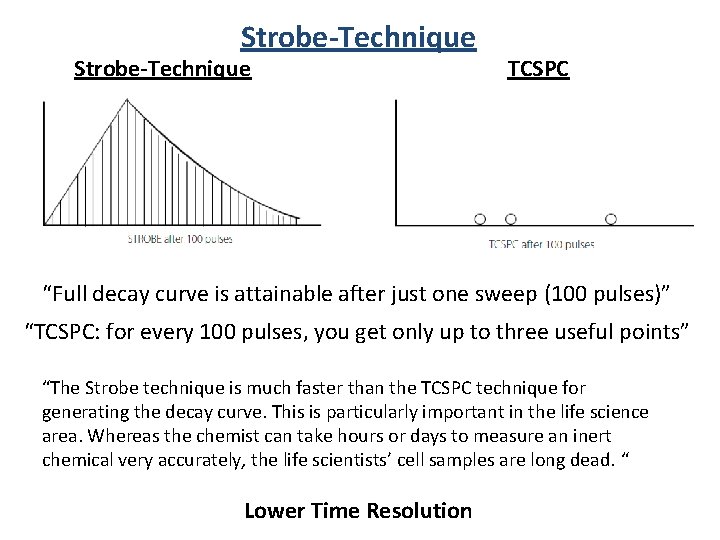
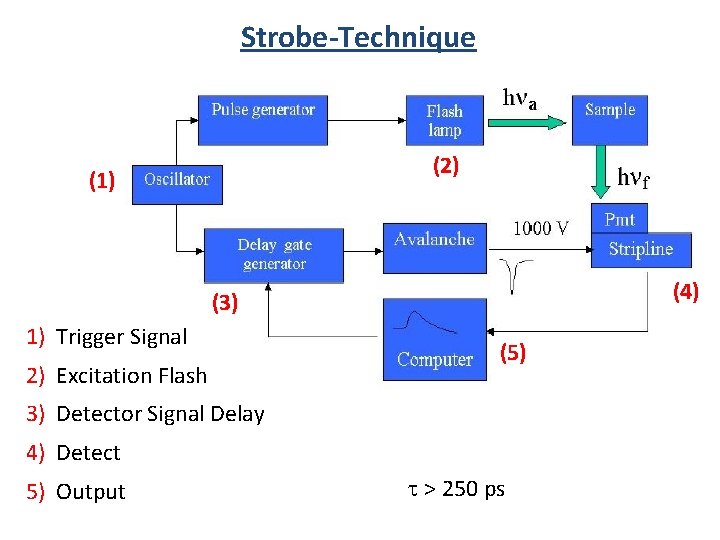
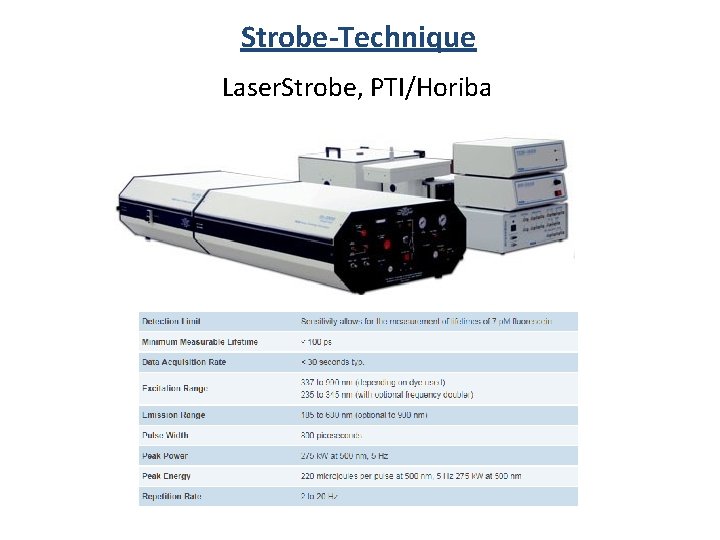
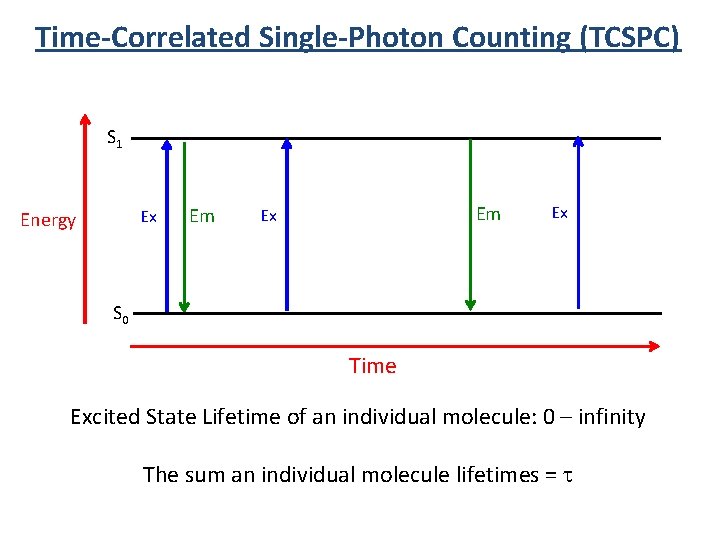
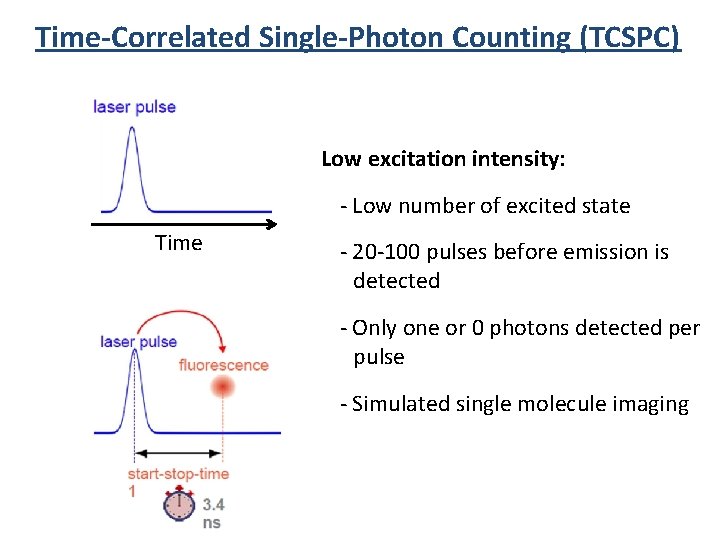
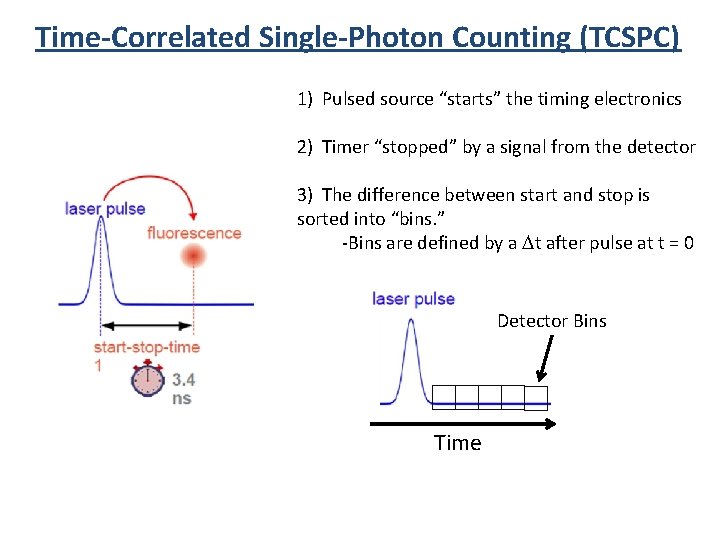
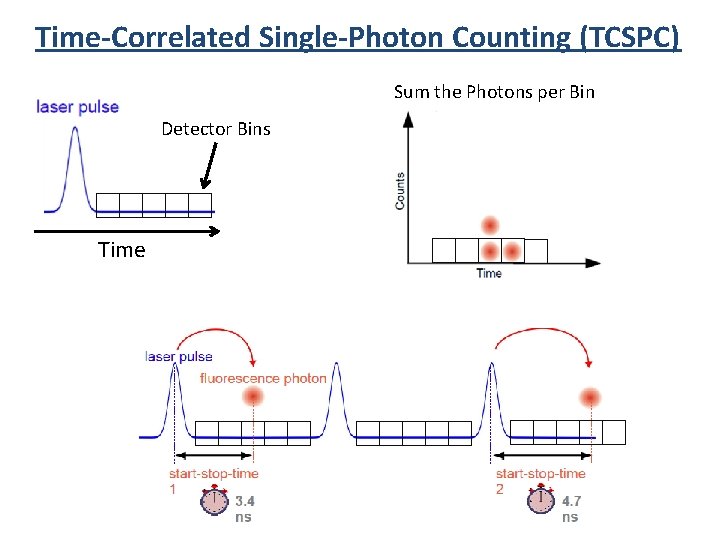
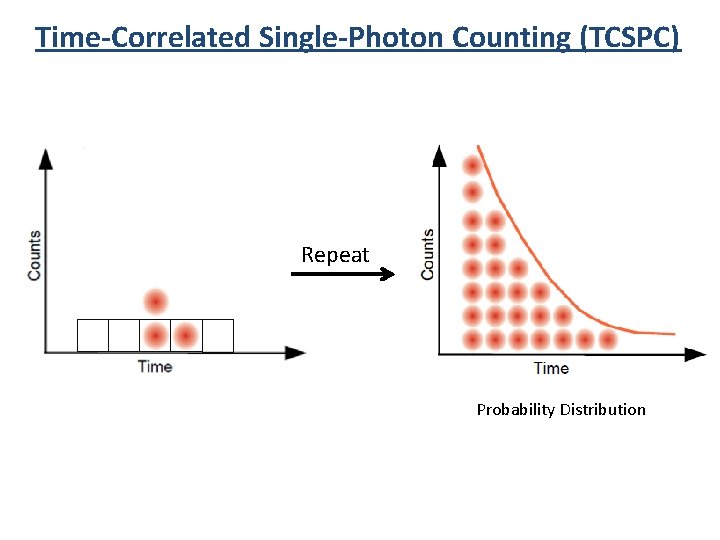
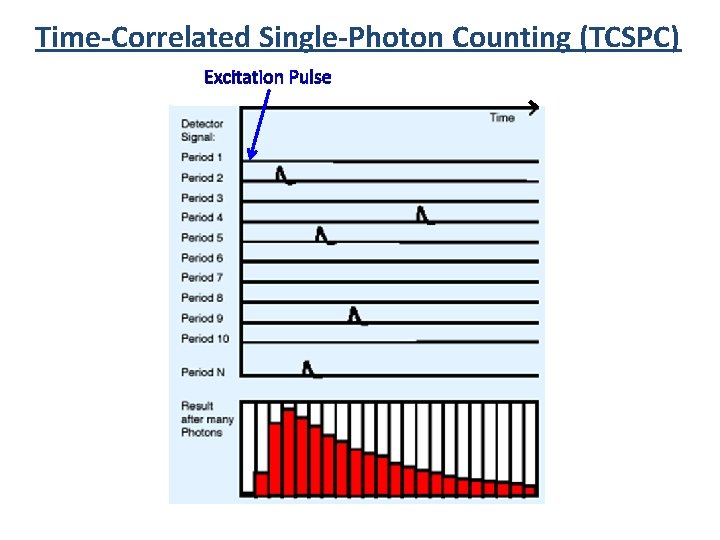
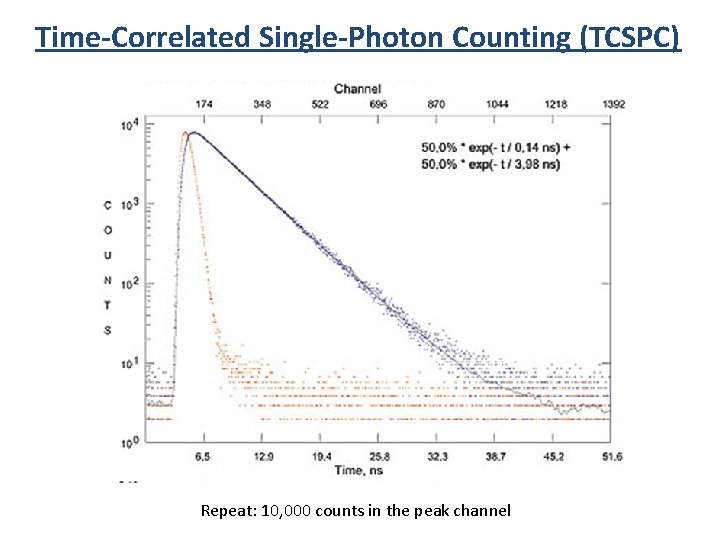
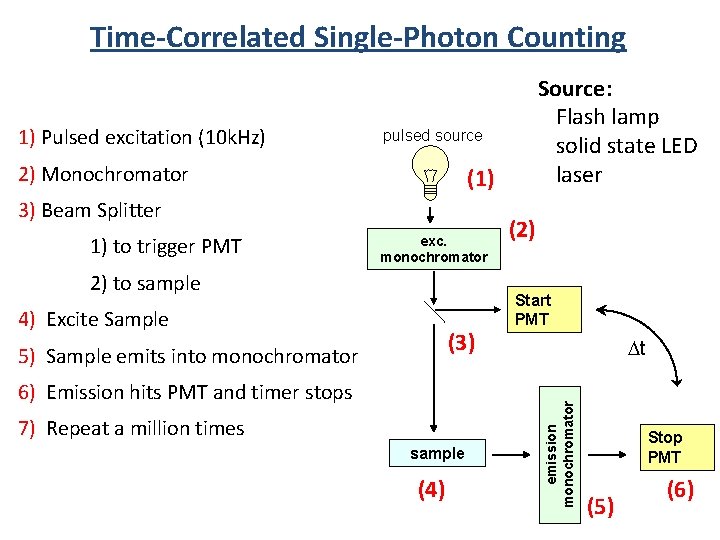
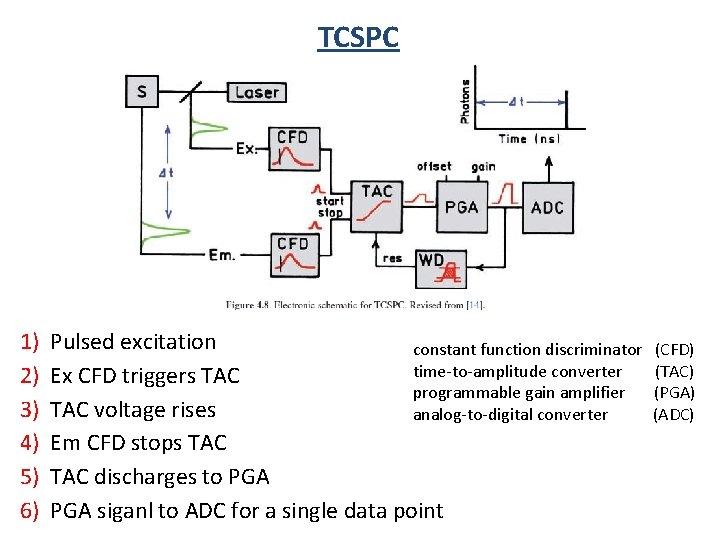
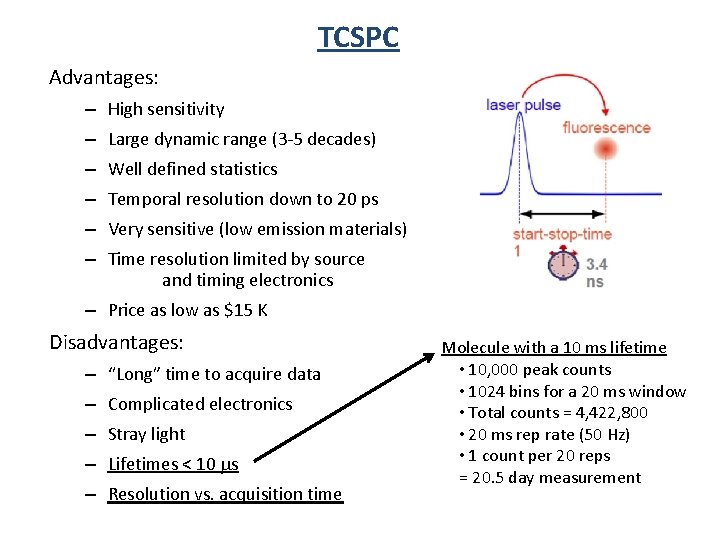
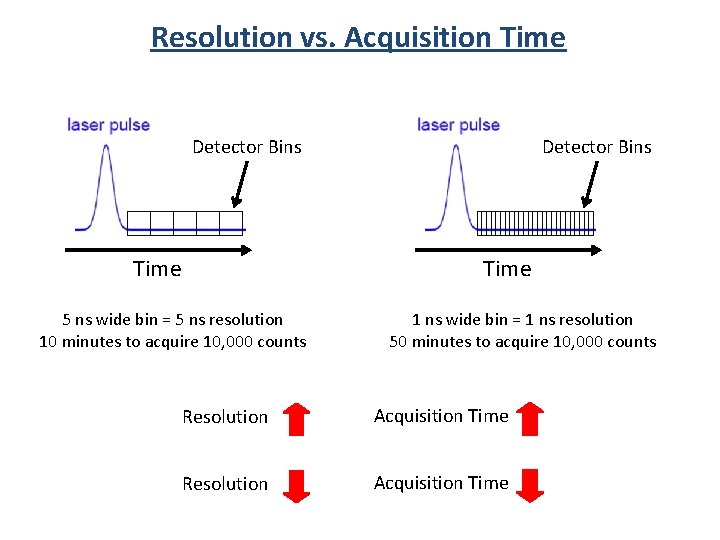
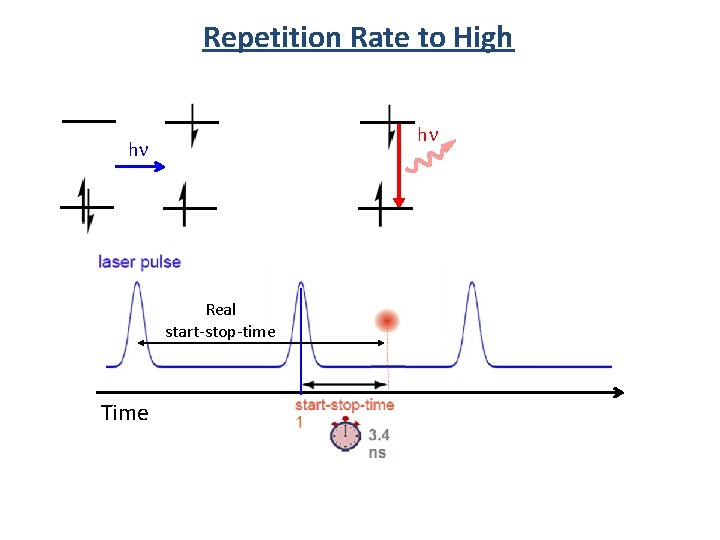
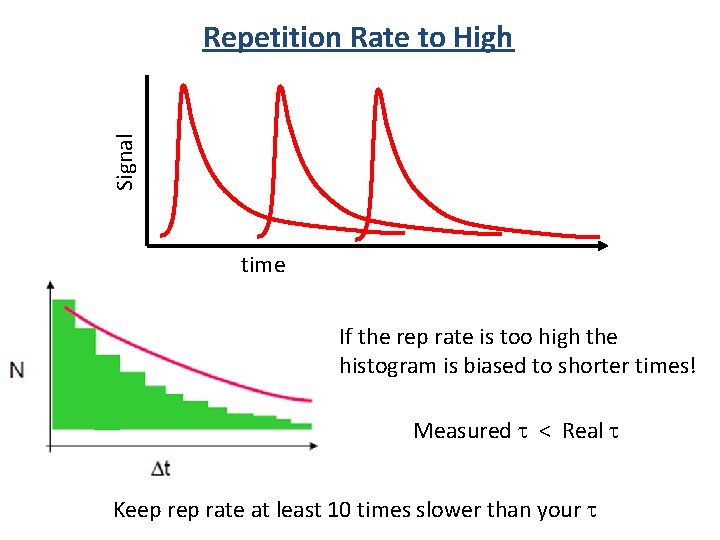
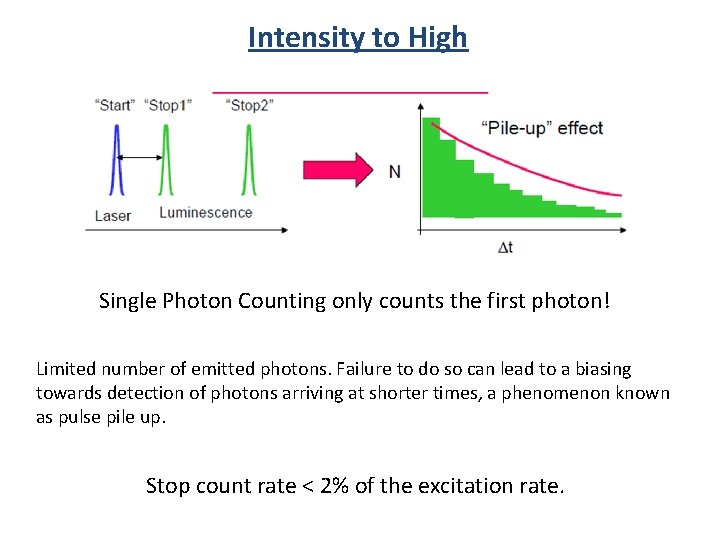
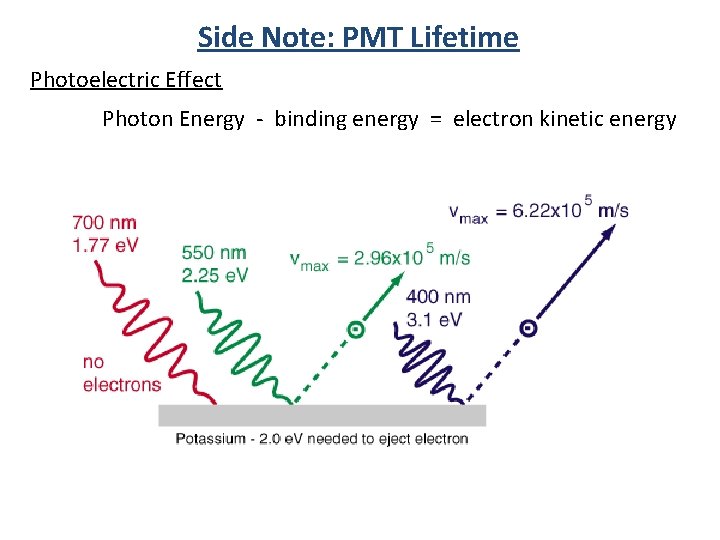
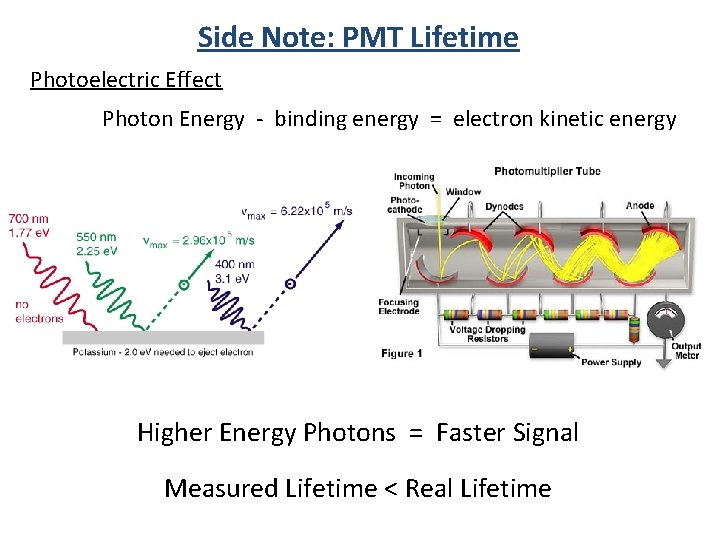
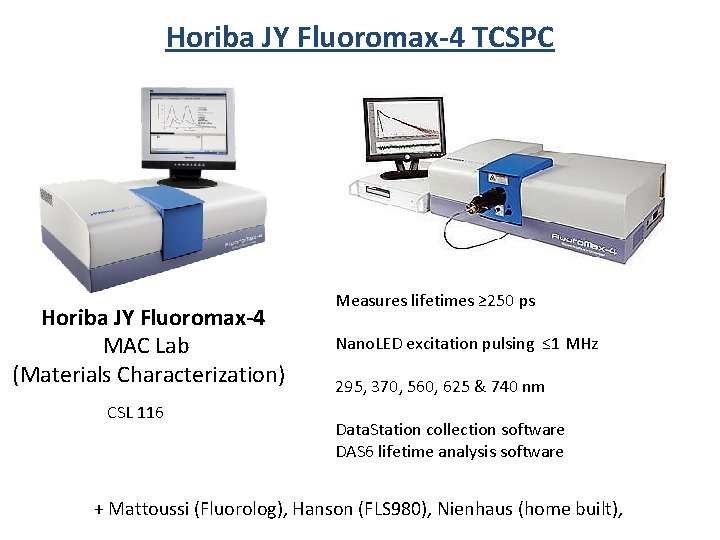
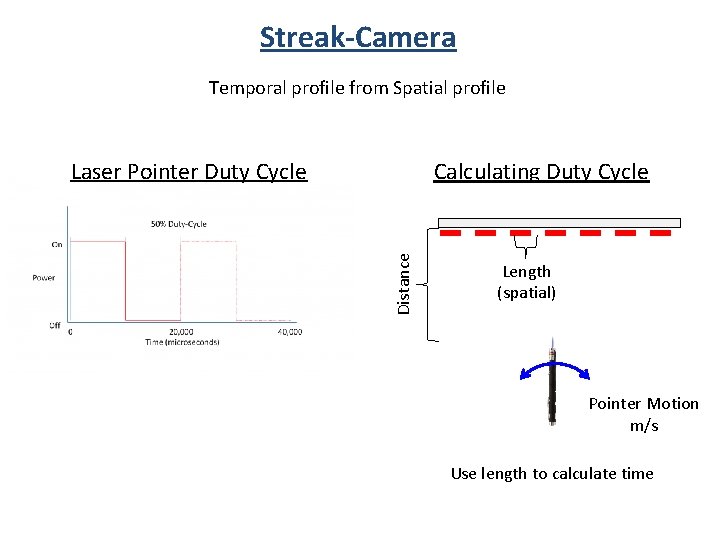
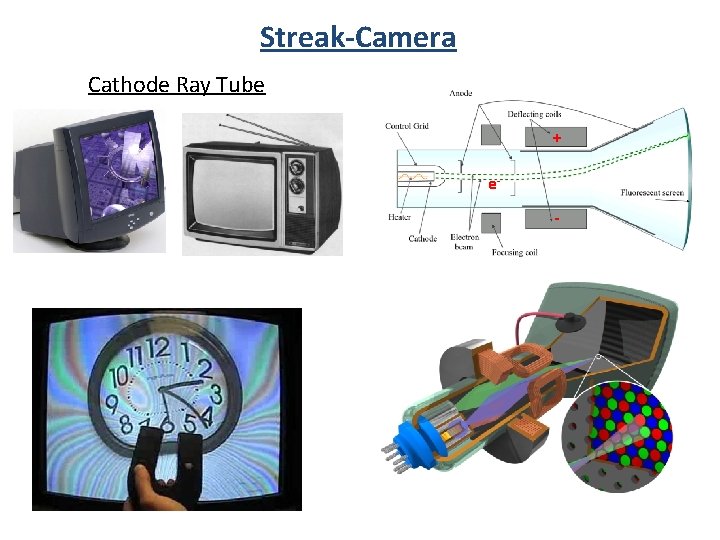
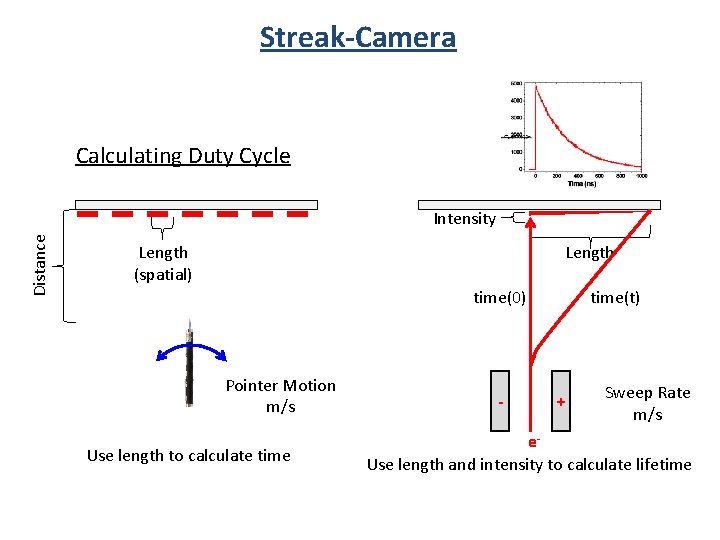
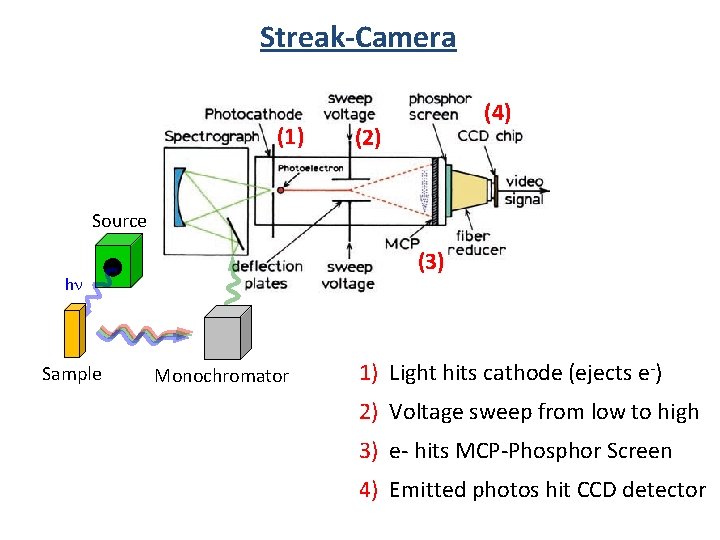
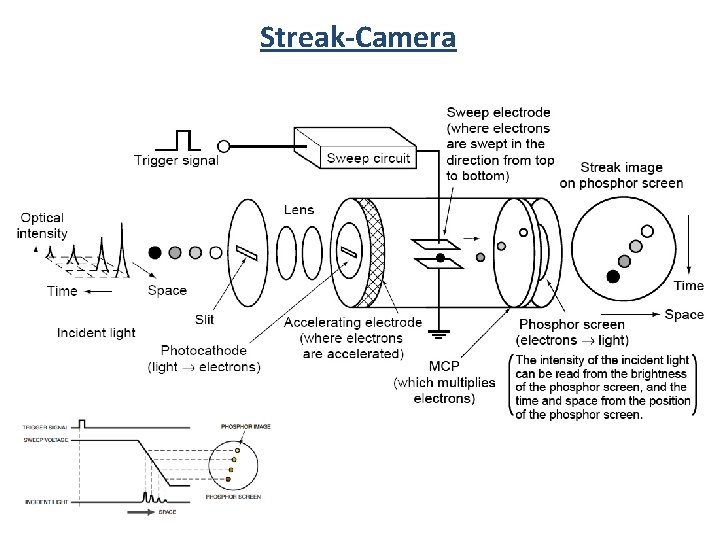
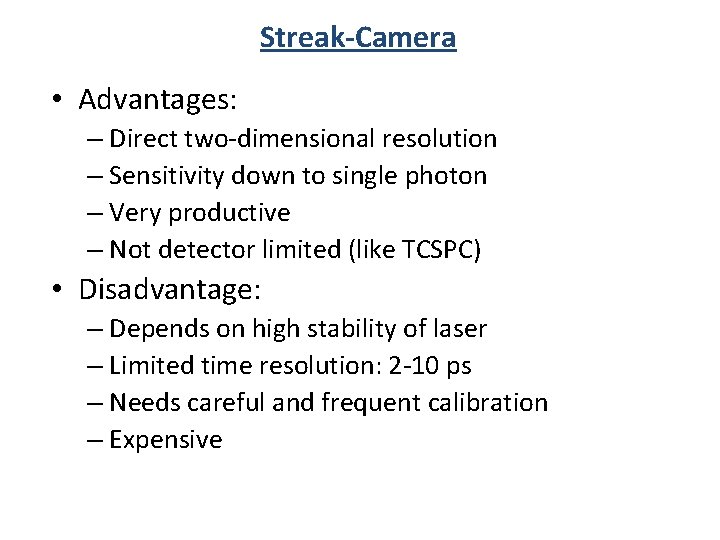
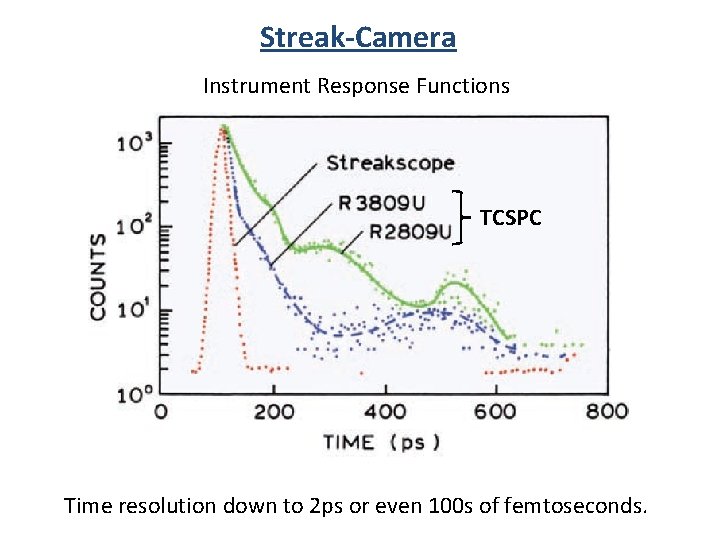
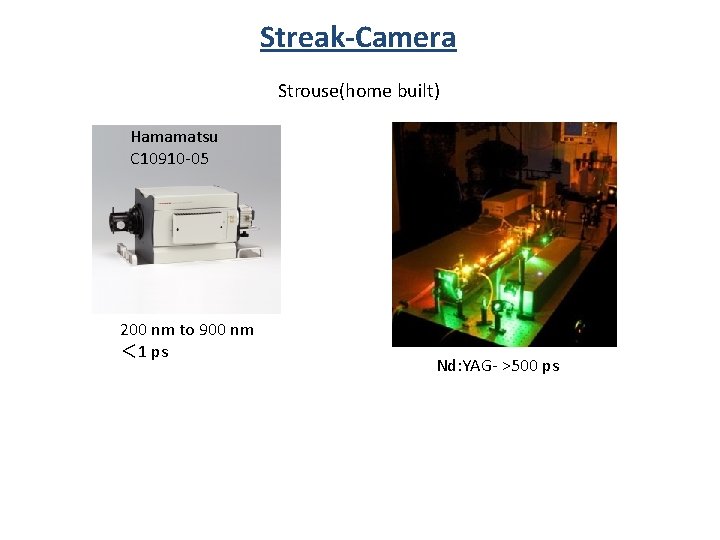
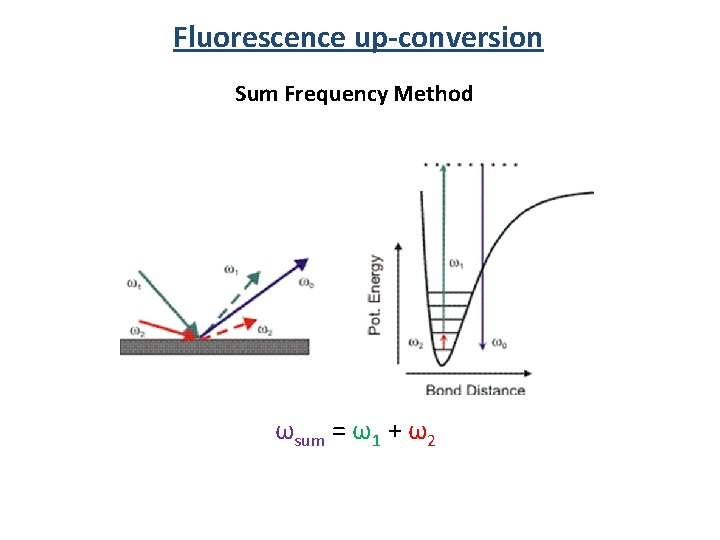
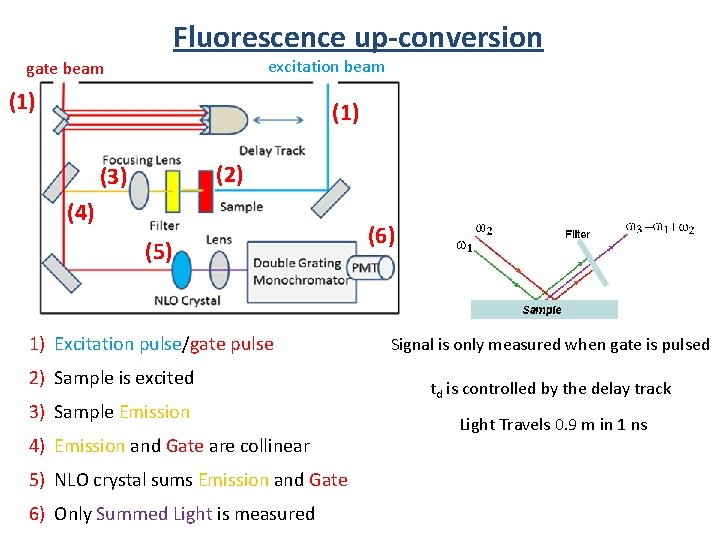
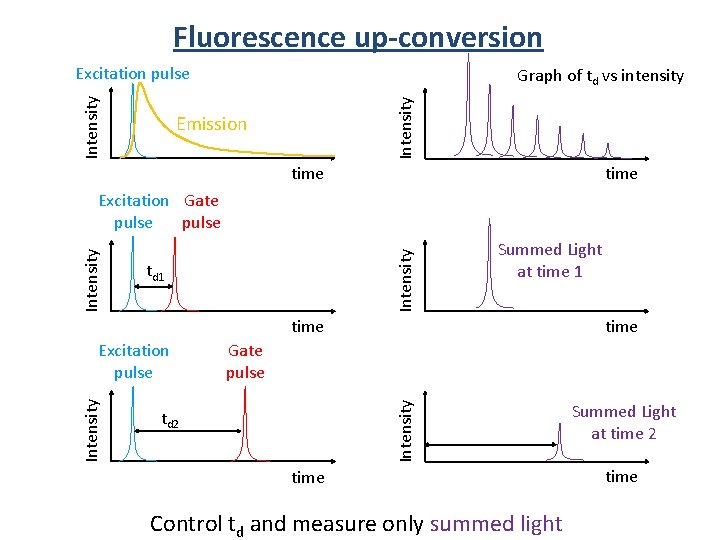
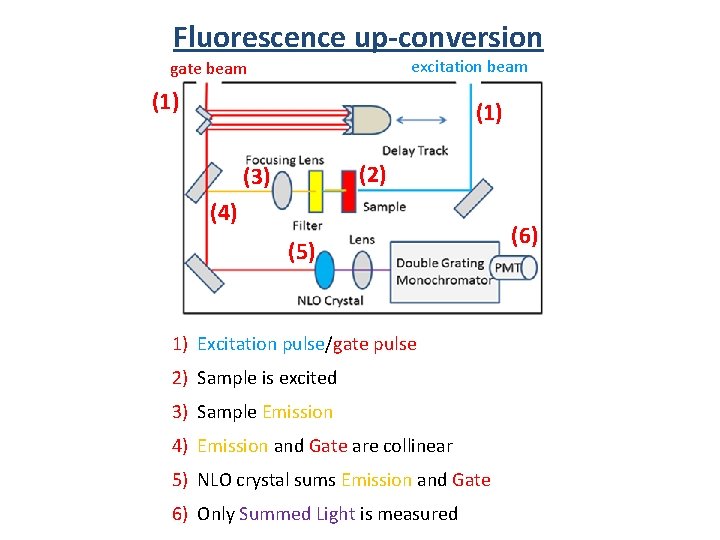
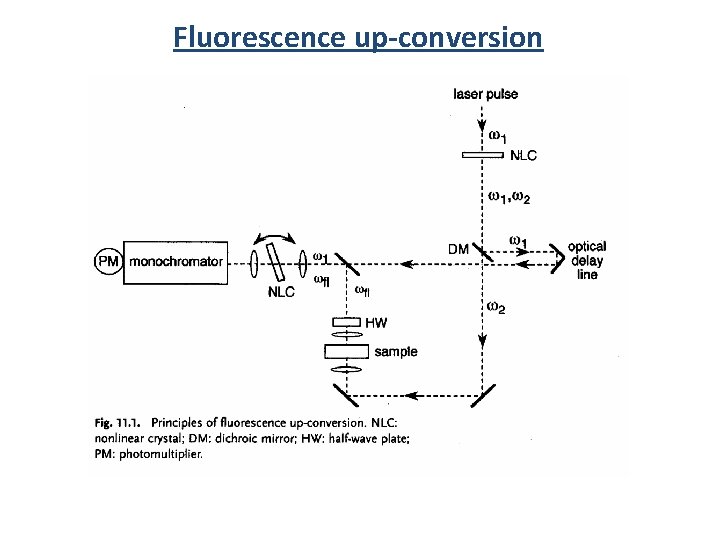
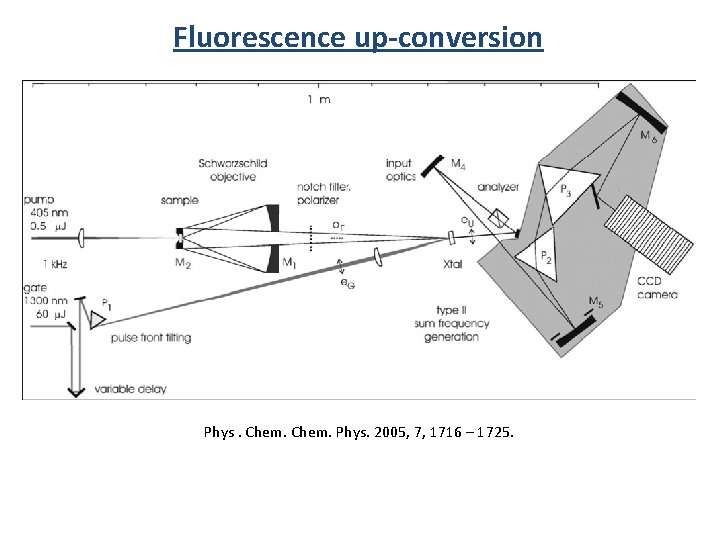
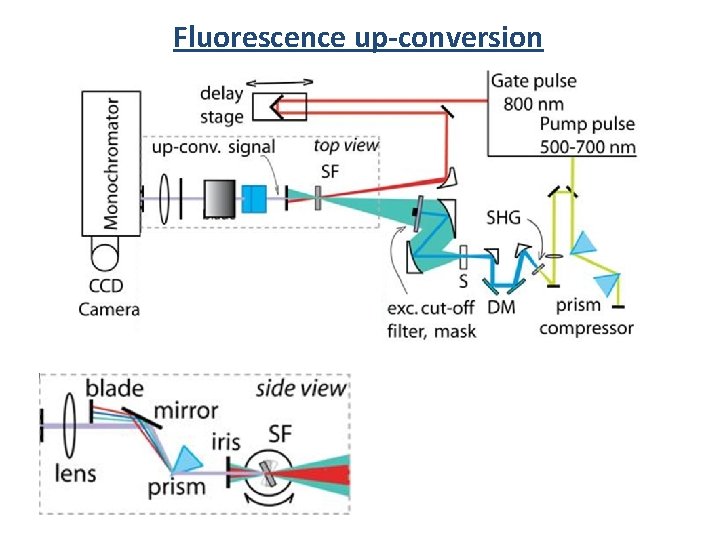
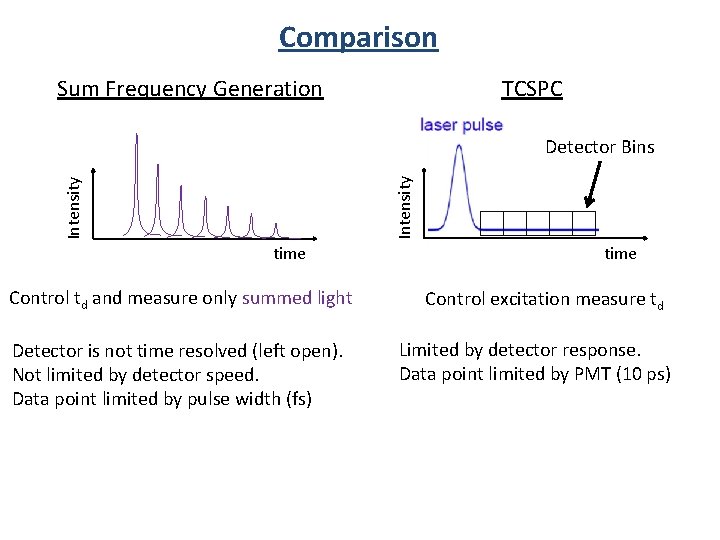
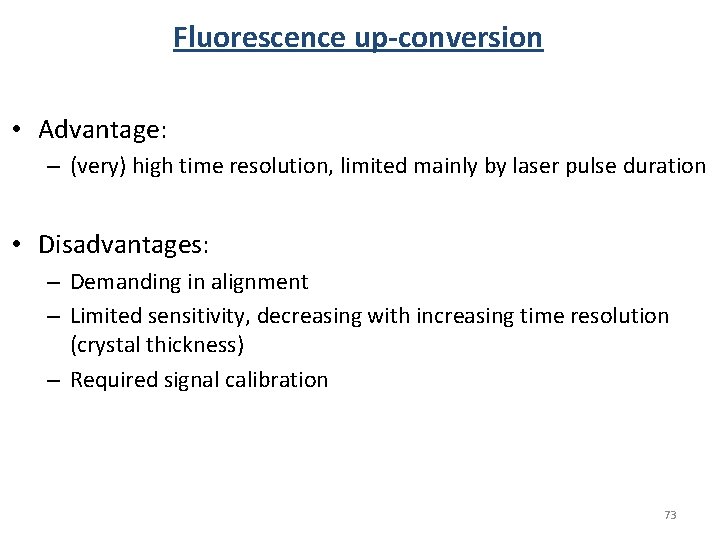
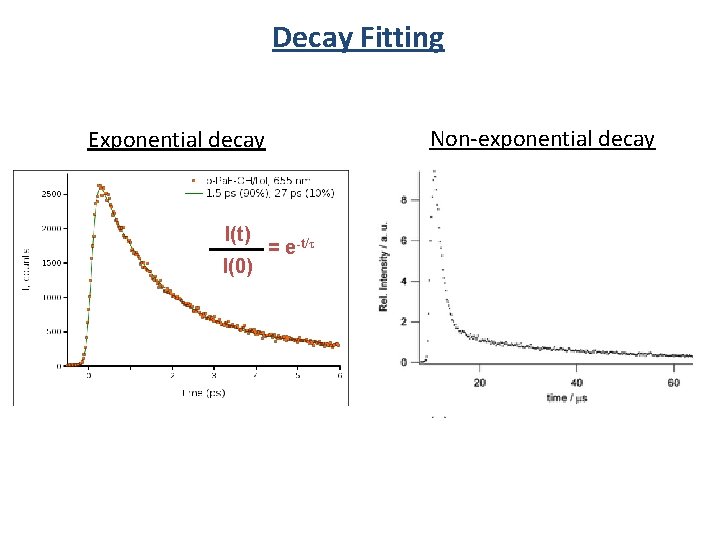
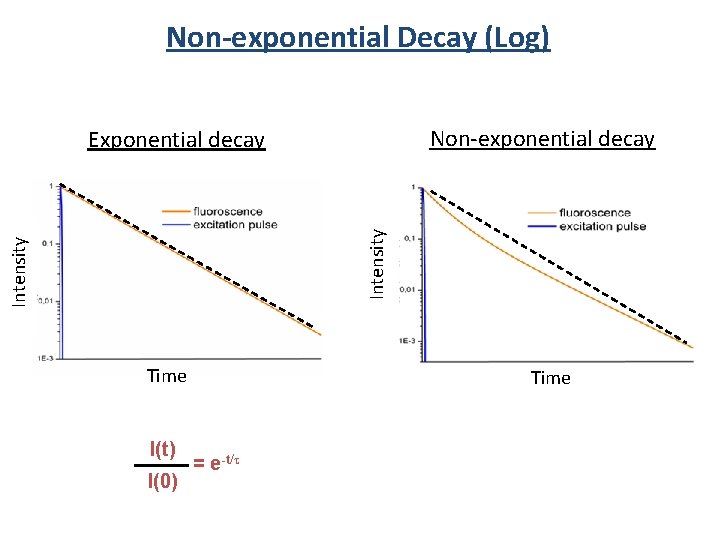
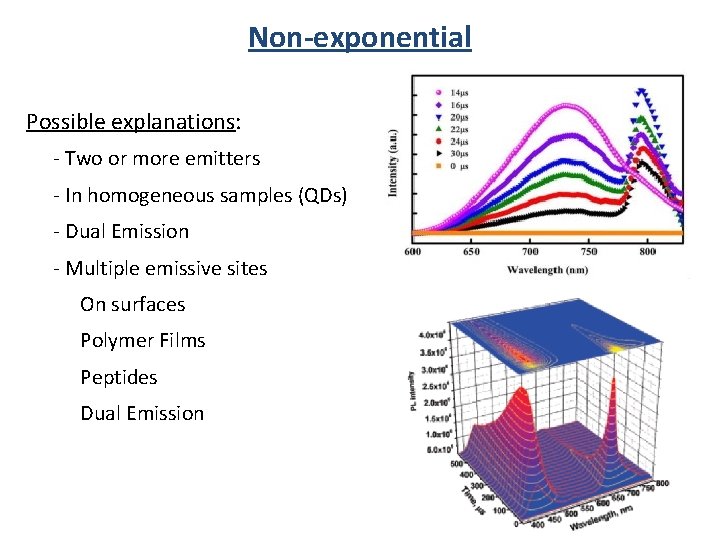
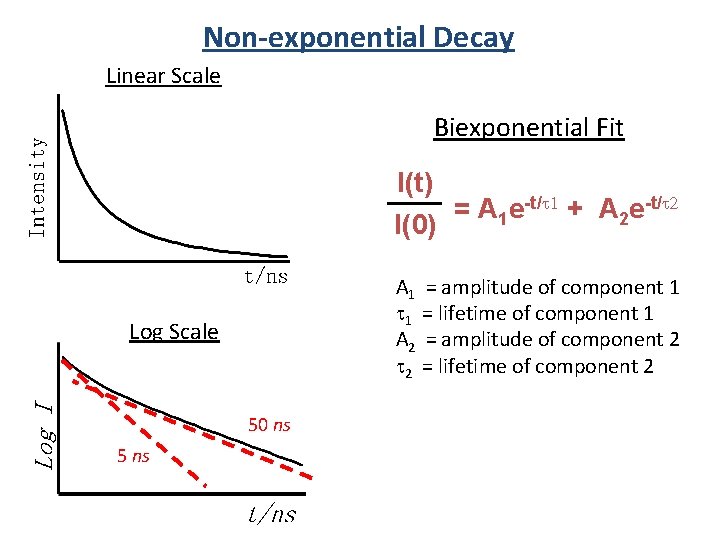
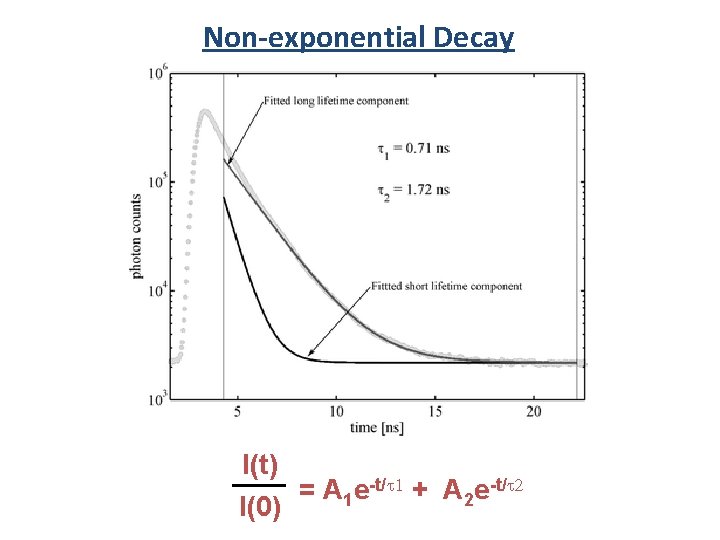
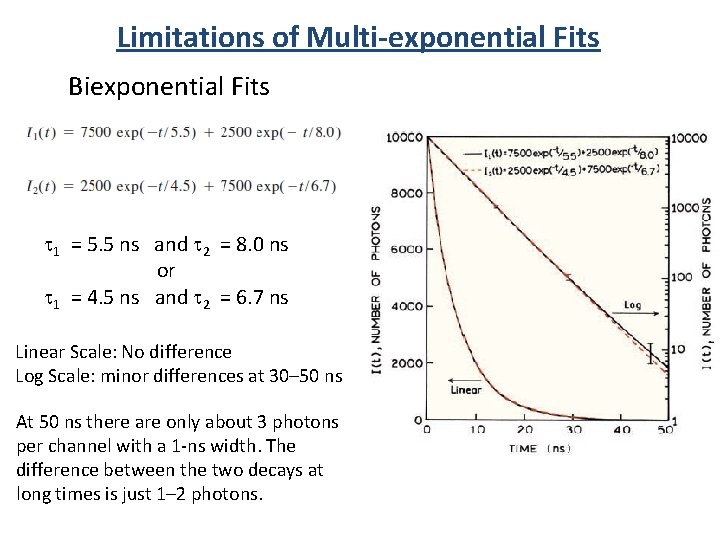
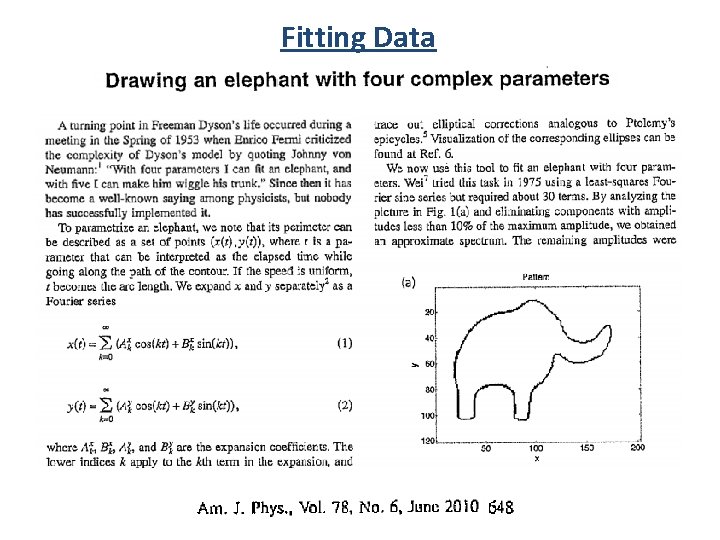
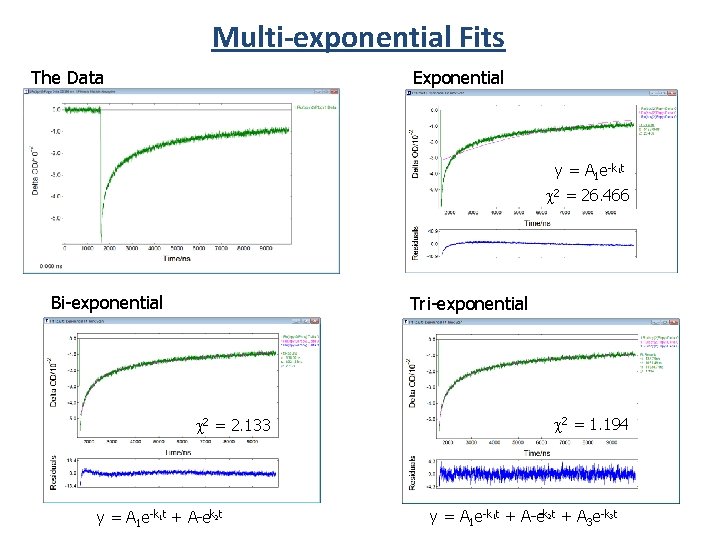
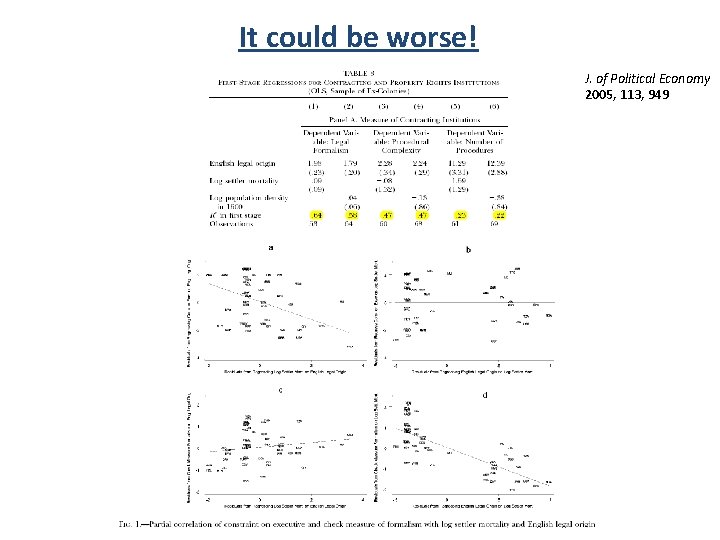
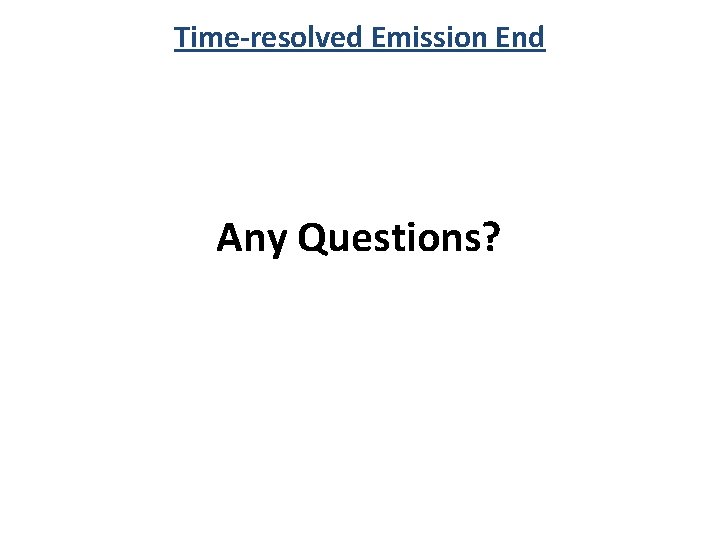
- Slides: 83
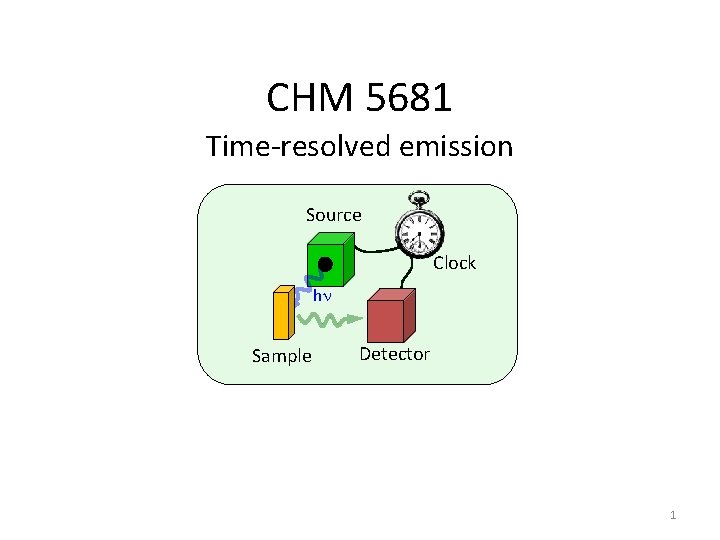
CHM 5681 Time-resolved emission Source Clock hn Sample Detector 1
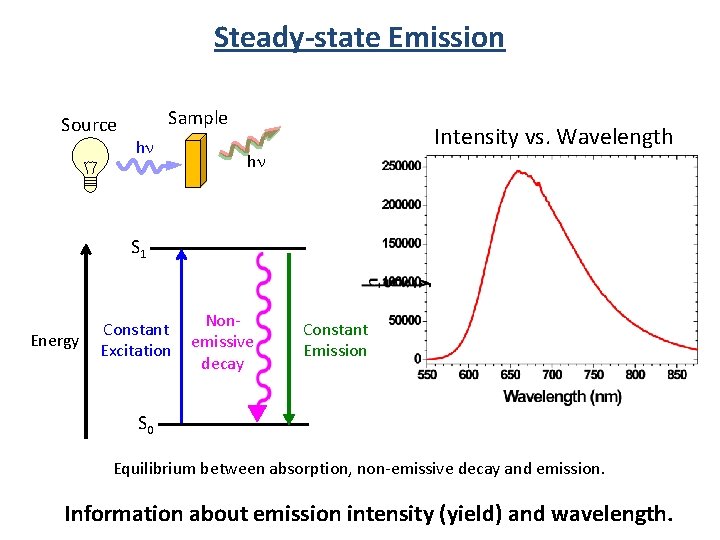
Steady-state Emission Source Sample hn Intensity vs. Wavelength hn S 1 Energy Constant Excitation Nonemissive decay Constant Emission S 0 Equilibrium between absorption, non-emissive decay and emission. Information about emission intensity (yield) and wavelength.
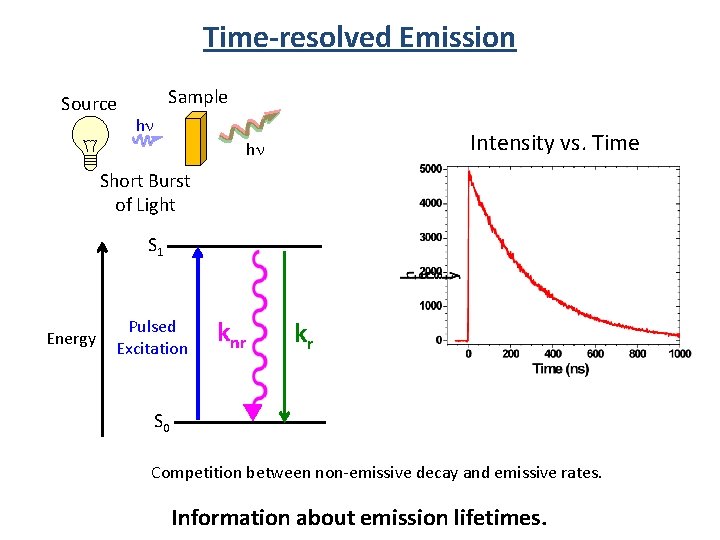
Time-resolved Emission Source Sample hn Intensity vs. Time hn Short Burst of Light S 1 Energy Pulsed Excitation knr kr S 0 Competition between non-emissive decay and emissive rates. Information about emission lifetimes.
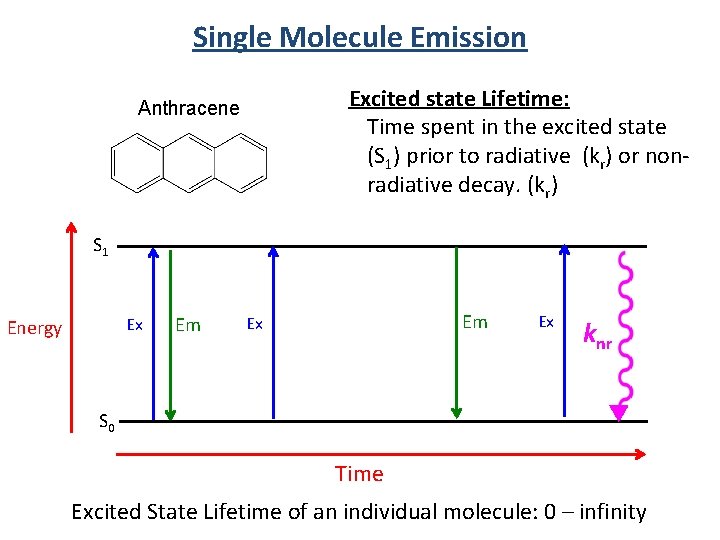
Single Molecule Emission Excited state Lifetime: Time spent in the excited state (S 1) prior to radiative (kr) or nonradiative decay. (kr) Anthracene S 1 Ex Energy Em Em Ex Ex knr S 0 Time Excited State Lifetime of an individual molecule: 0 – infinity
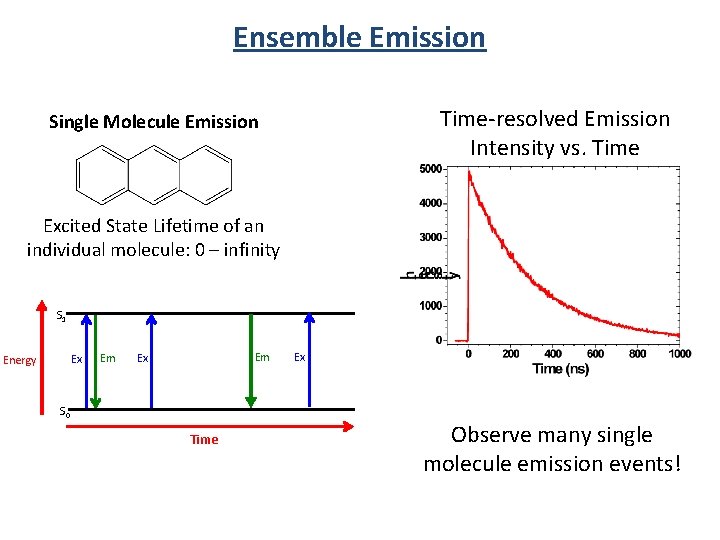
Ensemble Emission Time-resolved Emission Intensity vs. Time Single Molecule Emission Excited State Lifetime of an individual molecule: 0 – infinity S 1 Ex Energy Em Em Ex S 0 Time Ex Observe many single molecule emission events!
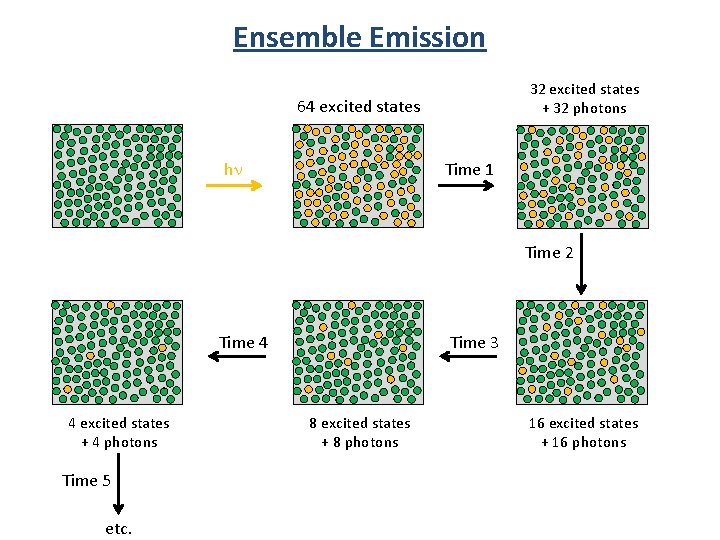
Ensemble Emission 32 excited states + 32 photons 64 excited states Time 1 hn Time 2 Time 4 4 excited states + 4 photons Time 5 etc. Time 3 8 excited states + 8 photons 16 excited states + 16 photons
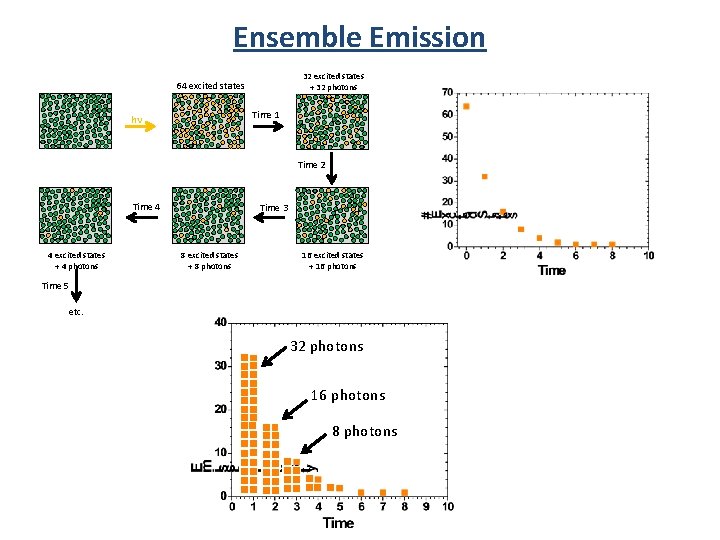
Ensemble Emission 32 excited states + 32 photons 64 excited states Time 1 hn Time 2 Time 4 4 excited states + 4 photons Time 3 8 excited states + 8 photons 16 excited states + 16 photons Time 5 etc. 32 photons 16 photons 8 photons
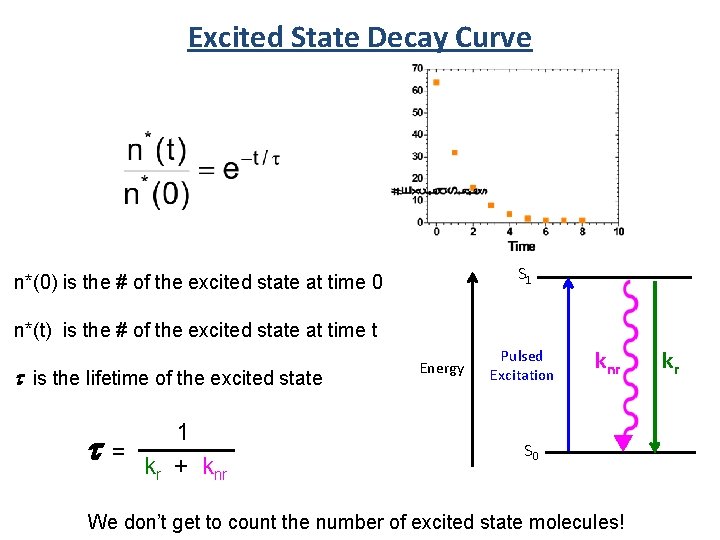
Excited State Decay Curve S 1 n*(0) is the # of the excited state at time 0 n*(t) is the # of the excited state at time t t is the lifetime of the excited state t 1 = kr + knr Energy Pulsed Excitation knr S 0 We don’t get to count the number of excited state molecules! kr
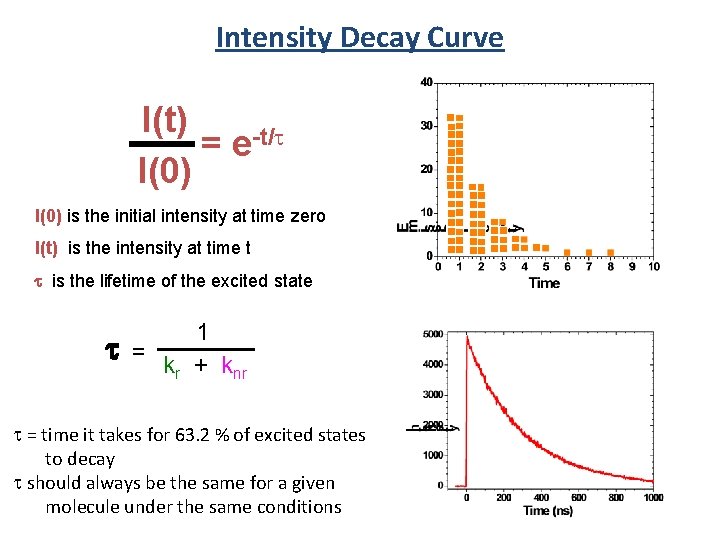
Intensity Decay Curve I(t) = e-t/ I(0) is the initial intensity at time zero I(t) is the intensity at time t t is the lifetime of the excited state t 1 = kr + knr = time it takes for 63. 2 % of excited states to decay should always be the same for a given molecule under the same conditions
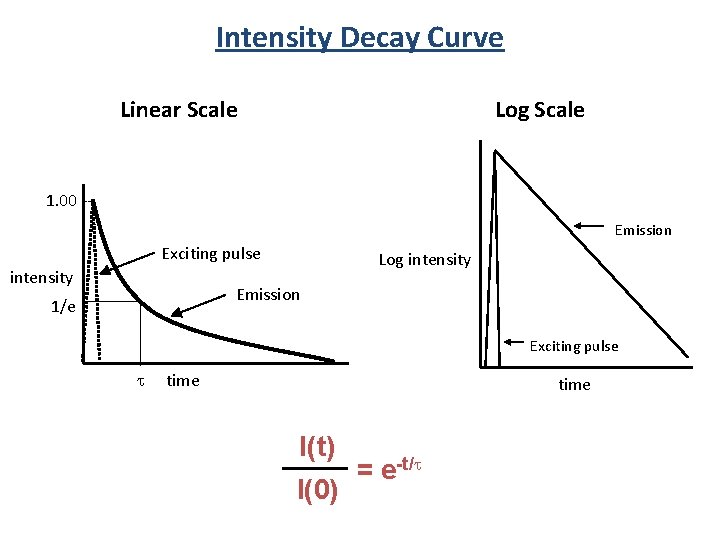
Intensity Decay Curve Linear Scale Log Scale 1. 00 -Emission Exciting pulse intensity Log intensity Emission 1/e Exciting pulse time I(t) I(0) = e-t/
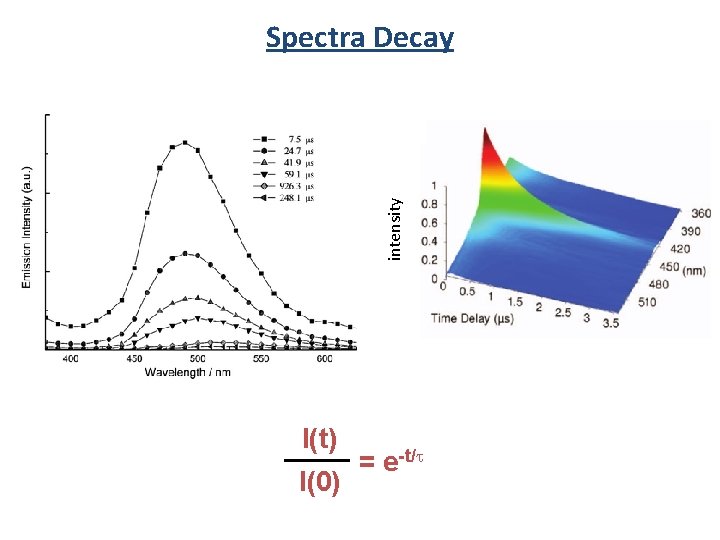
intensity Spectra Decay I(t) I(0) = e-t/
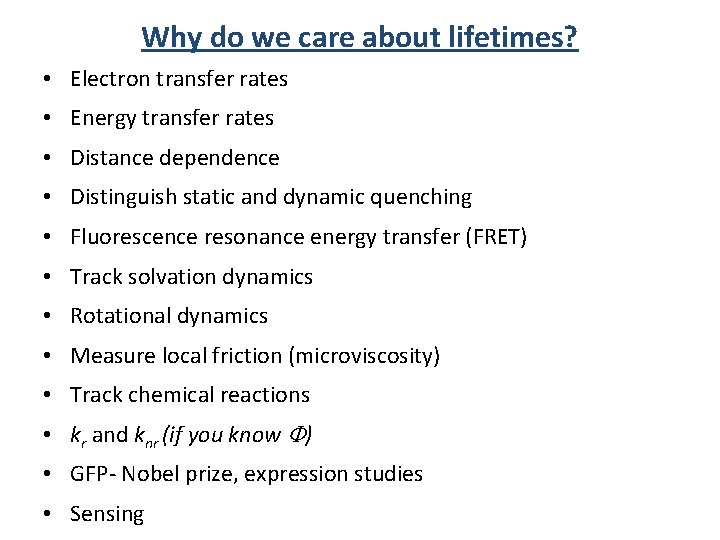
Why do we care about lifetimes? • Electron transfer rates • Energy transfer rates • Distance dependence • Distinguish static and dynamic quenching • Fluorescence resonance energy transfer (FRET) • Track solvation dynamics • Rotational dynamics • Measure local friction (microviscosity) • Track chemical reactions • kr and knr (if you know F) • GFP- Nobel prize, expression studies • Sensing
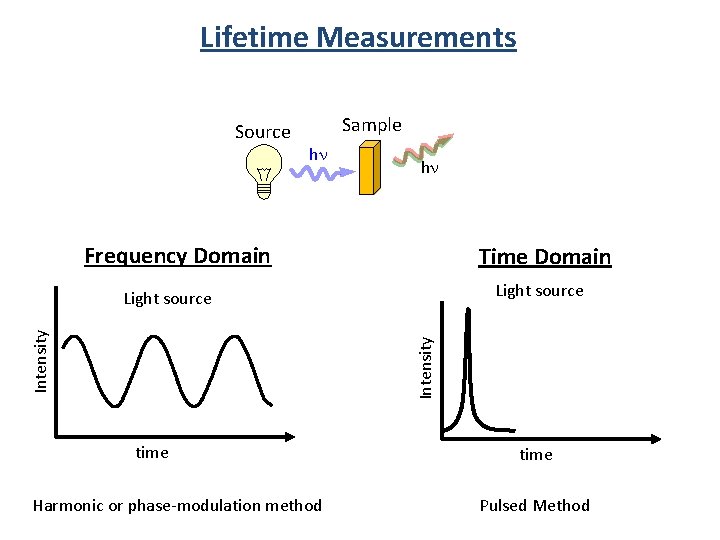
Lifetime Measurements Source Sample hn hn Frequency Domain Time Domain Light source Intensity Light source time Harmonic or phase-modulation method time Pulsed Method
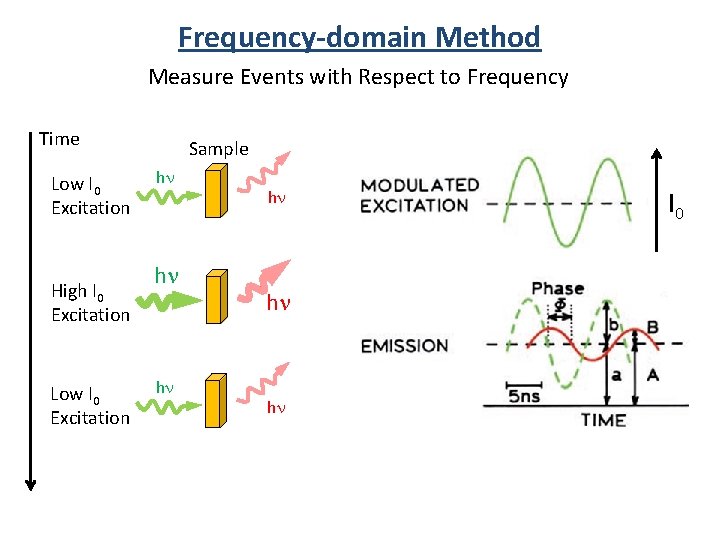
Frequency-domain Method Measure Events with Respect to Frequency Time Low I 0 Excitation High I 0 Excitation Low I 0 Excitation Sample hn hn hn I 0
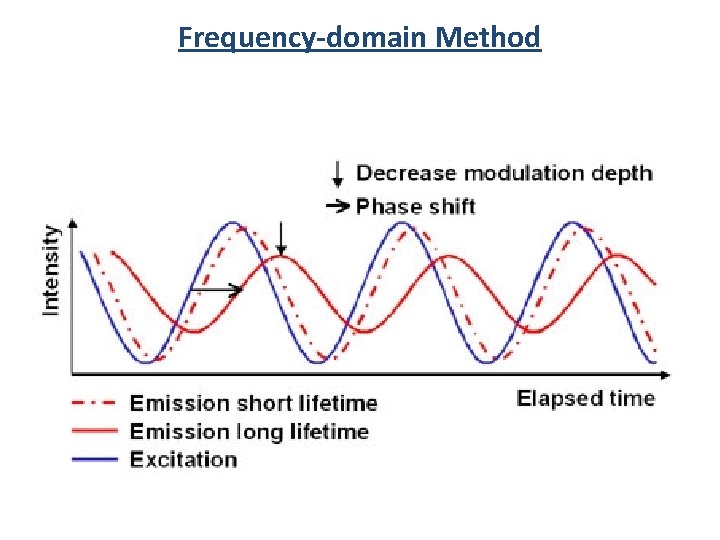
Frequency-domain Method
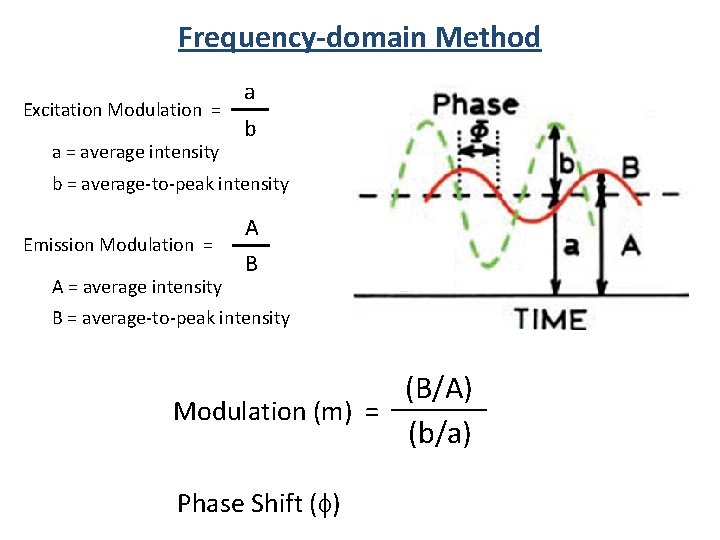
Frequency-domain Method Excitation Modulation = a = average intensity a b b = average-to-peak intensity Emission Modulation = A = average intensity A B B = average-to-peak intensity (B/A) Modulation (m) = (b/a) Phase Shift (f)
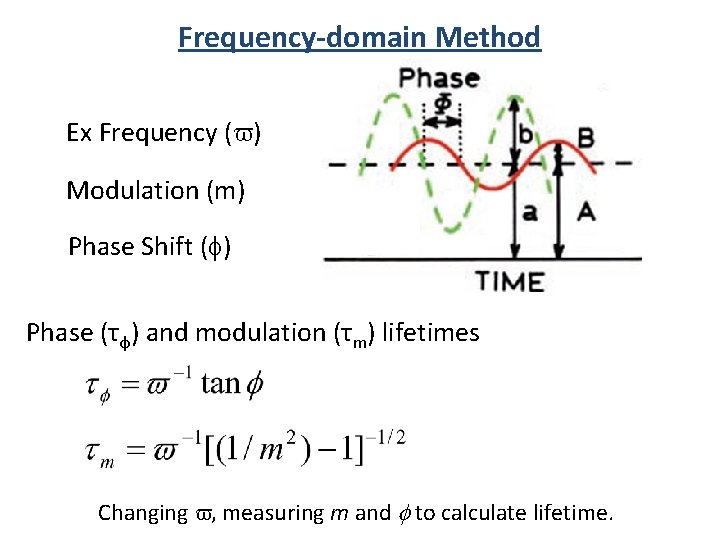
Frequency-domain Method Ex Frequency ( ) Modulation (m) Phase Shift (f) Phase (τφ) and modulation (τm) lifetimes Changing , measuring m and to calculate lifetime.
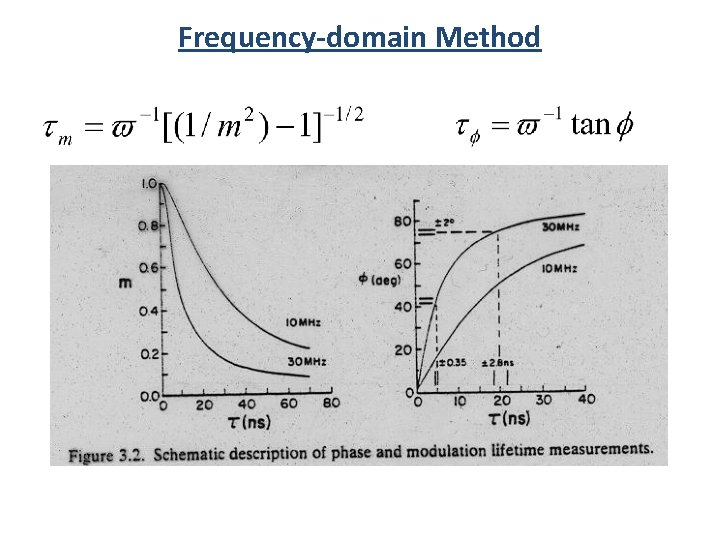
Frequency-domain Method
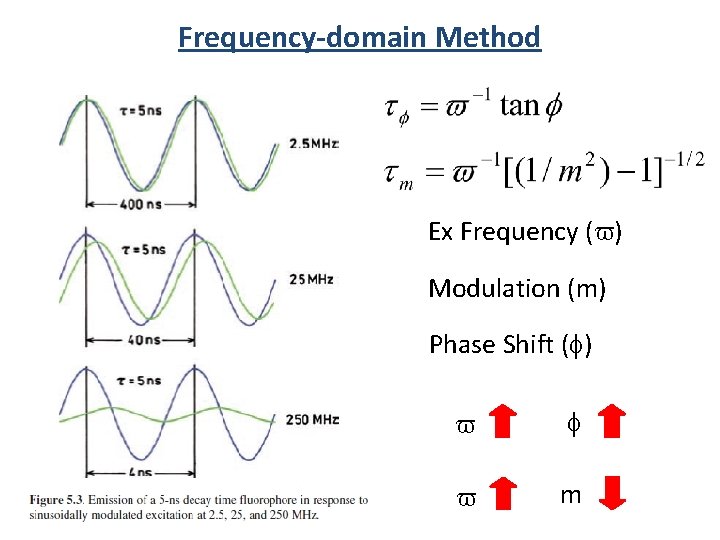
Frequency-domain Method Ex Frequency ( ) Modulation (m) Phase Shift (f) f m
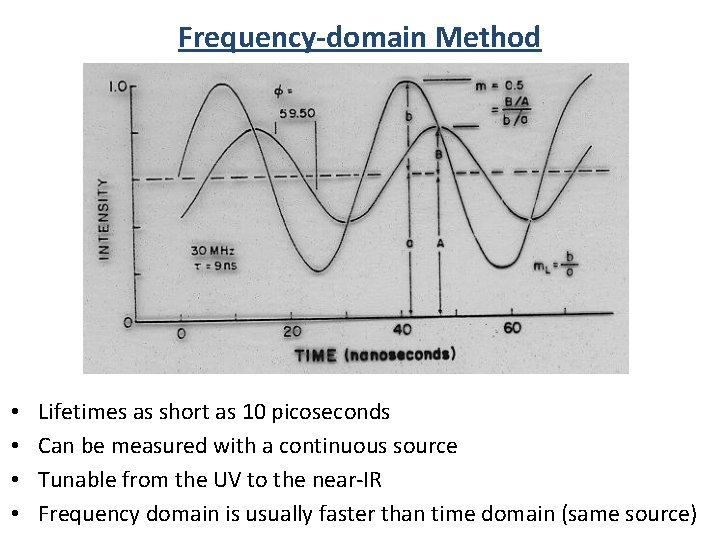
Frequency-domain Method • • Lifetimes as short as 10 picoseconds Can be measured with a continuous source Tunable from the UV to the near-IR Frequency domain is usually faster than time domain (same source)
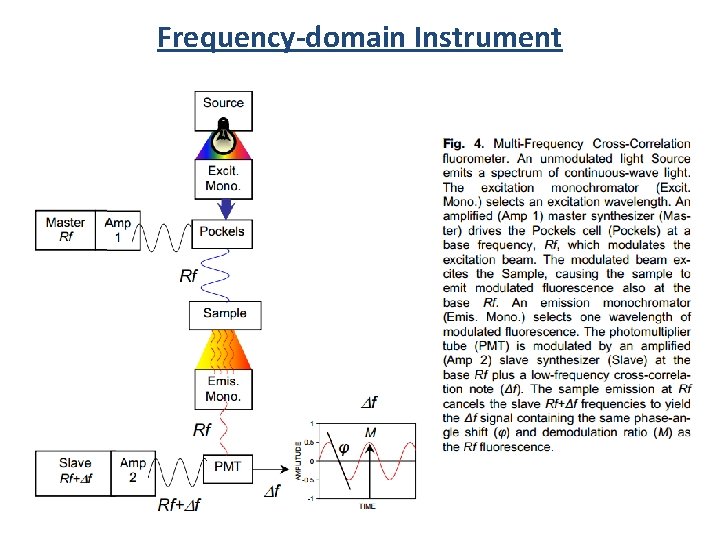
Frequency-domain Instrument
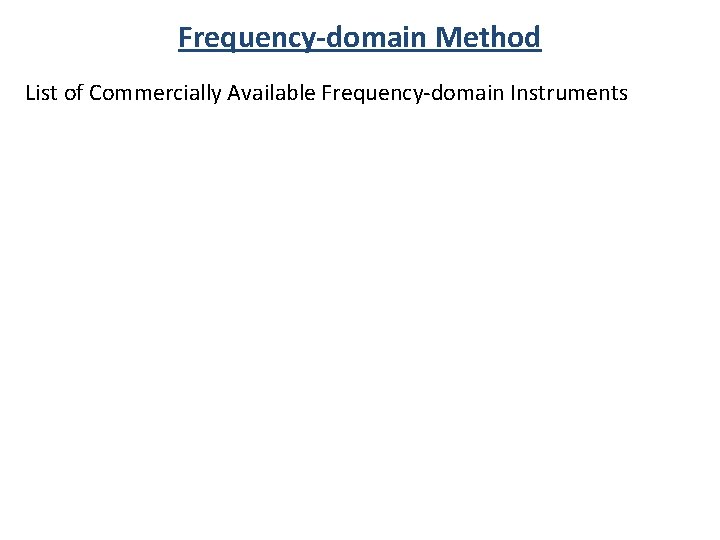
Frequency-domain Method List of Commercially Available Frequency-domain Instruments
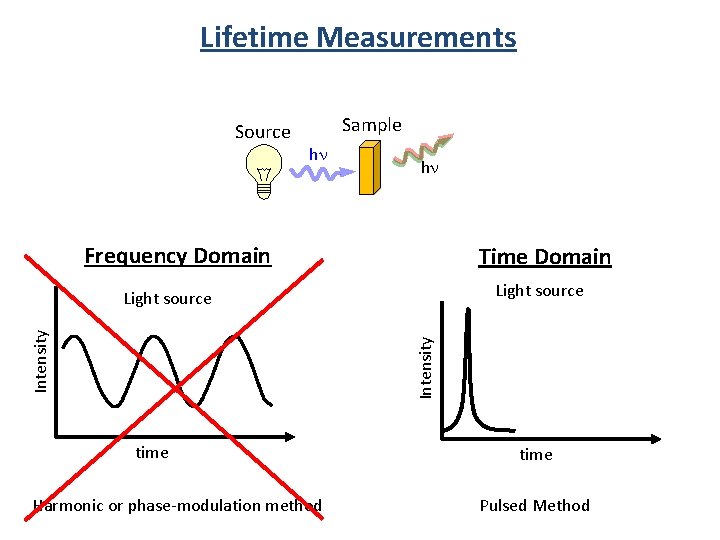
Lifetime Measurements Source Sample hn hn Frequency Domain Time Domain Light source Intensity Light source time Harmonic or phase-modulation method time Pulsed Method
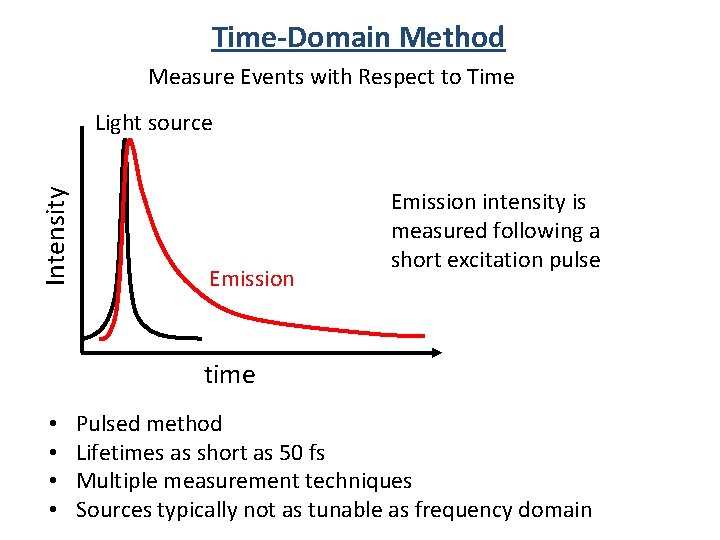
Time-Domain Method Measure Events with Respect to Time Intensity Light source Emission intensity is measured following a short excitation pulse time • • Pulsed method Lifetimes as short as 50 fs Multiple measurement techniques Sources typically not as tunable as frequency domain
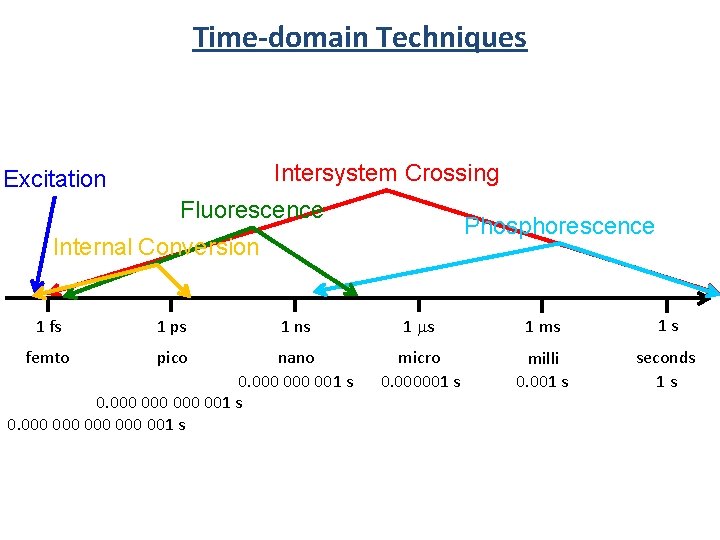
Time-domain Techniques Intersystem Crossing Excitation Fluorescence Phosphorescence Internal Conversion 1 fs 1 ps femto pico 1 ns nano 0. 000 000 001 s 0. 000 000 000 001 s 1 ms 1 s micro 0. 000001 s milli 0. 001 s seconds 1 s
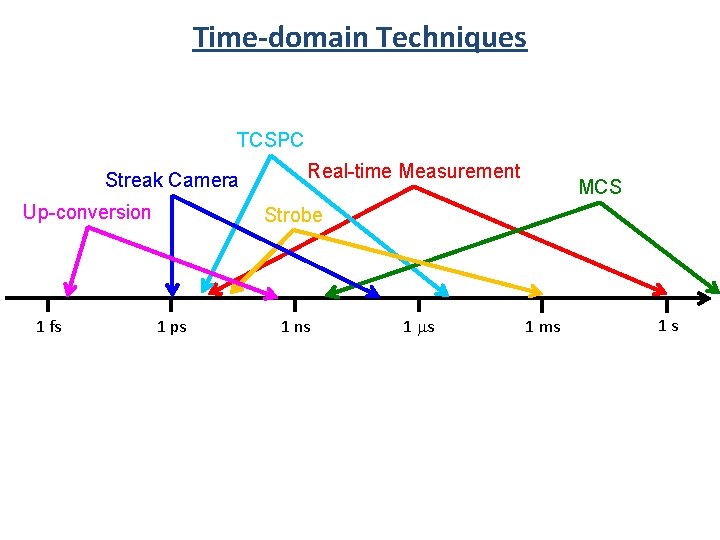
Time-domain Techniques TCSPC Streak Camera Up-conversion 1 fs Real-time Measurement MCS Strobe 1 ps 1 ns 1 ms 1 s
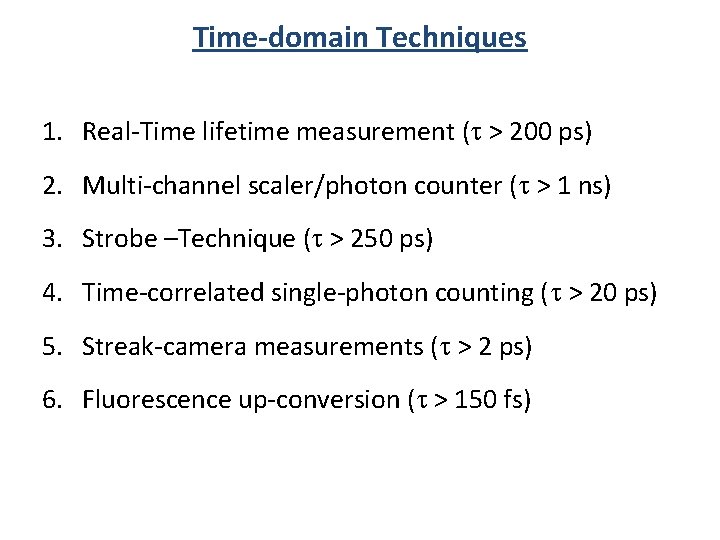
Time-domain Techniques 1. Real-Time lifetime measurement ( > 200 ps) 2. Multi-channel scaler/photon counter ( > 1 ns) 3. Strobe –Technique ( > 250 ps) 4. Time-correlated single-photon counting ( > 20 ps) 5. Streak-camera measurements ( > 2 ps) 6. Fluorescence up-conversion ( > 150 fs)
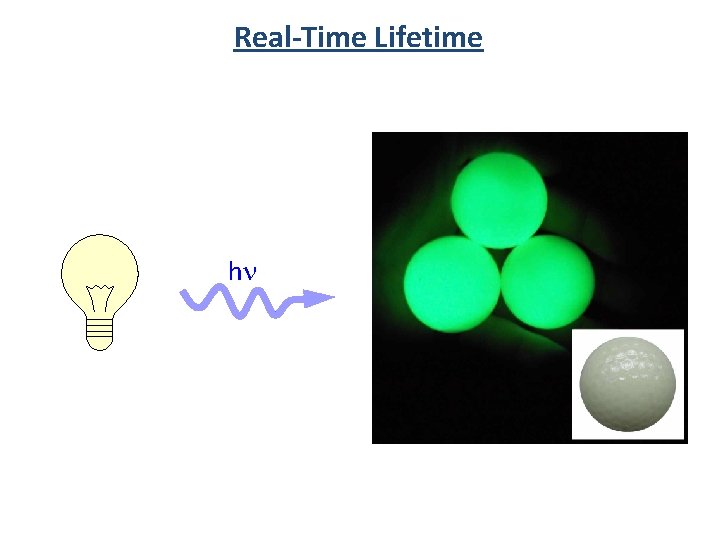
Real-Time Lifetime hn
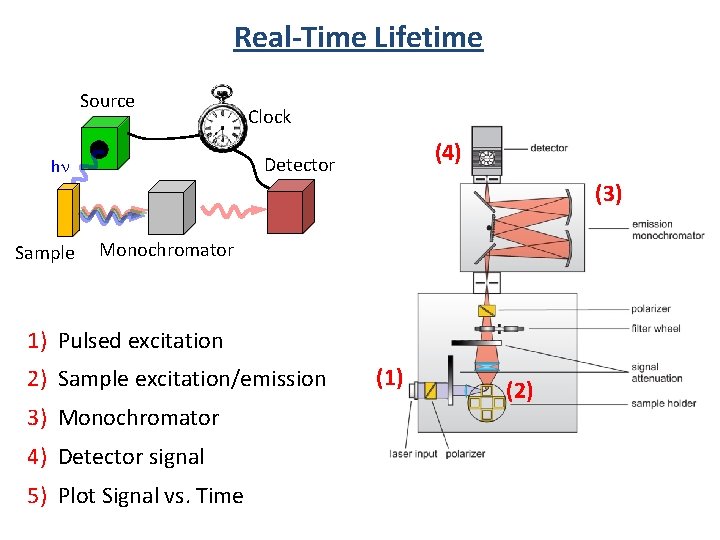
Real-Time Lifetime Source Clock (4) Detector hn (3) Sample Monochromator 1) Pulsed excitation 2) Sample excitation/emission 3) Monochromator 4) Detector signal 5) Plot Signal vs. Time (1) (2)
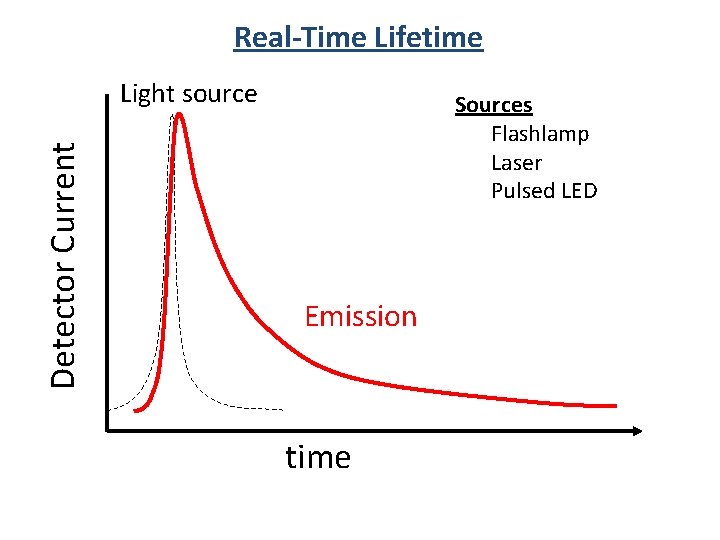
Real-Time Lifetime Detector Current Light source Sources Flashlamp Laser Pulsed LED Emission time
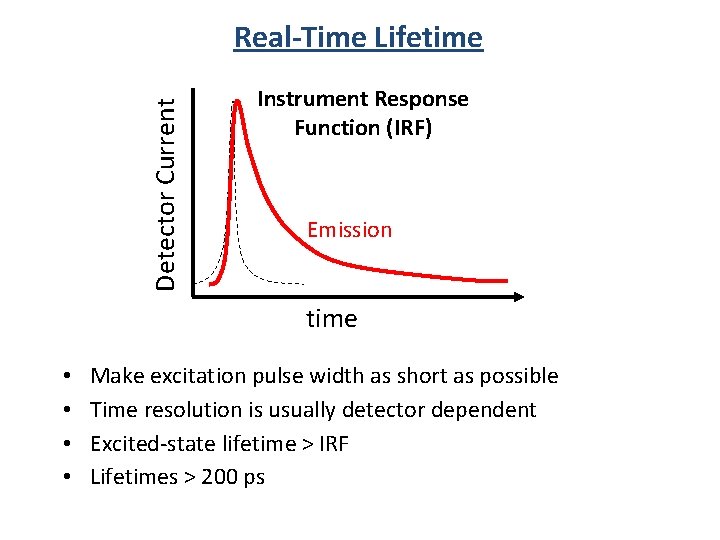
Detector Current Real-Time Lifetime Instrument Response Function (IRF) Emission time • • Make excitation pulse width as short as possible Time resolution is usually detector dependent Excited-state lifetime > IRF Lifetimes > 200 ps
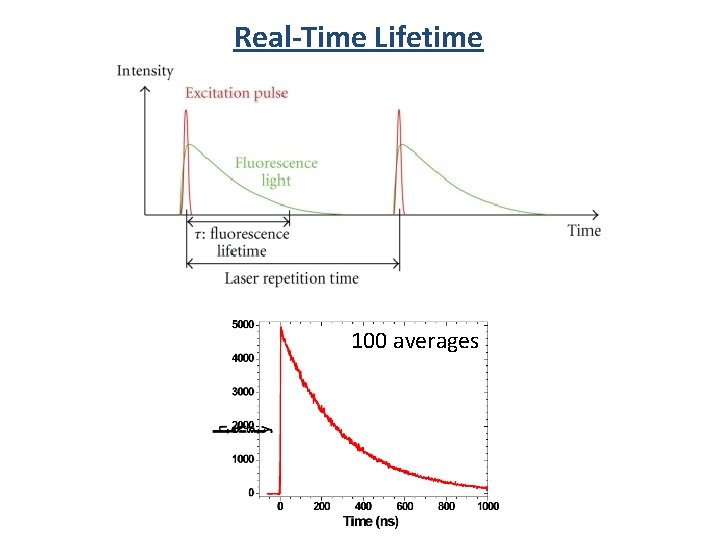
Real-Time Lifetime 100 averages
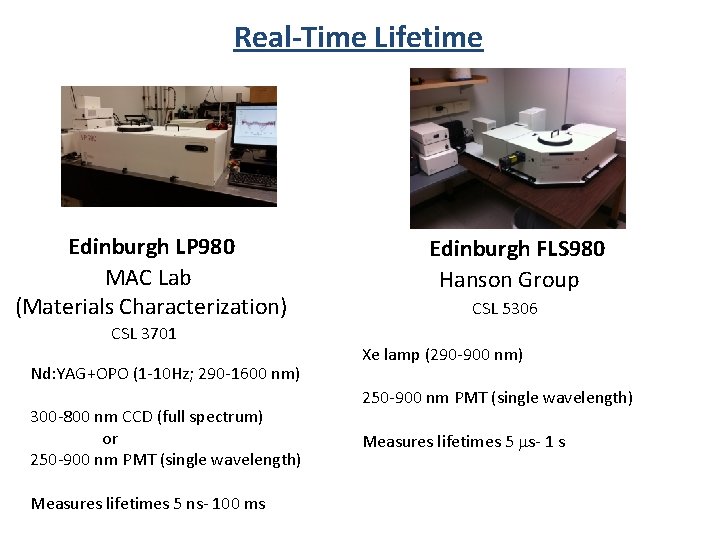
Real-Time Lifetime Edinburgh LP 980 MAC Lab (Materials Characterization) CSL 3701 Nd: YAG+OPO (1 -10 Hz; 290 -1600 nm) 300 -800 nm CCD (full spectrum) or 250 -900 nm PMT (single wavelength) Measures lifetimes 5 ns- 100 ms Edinburgh FLS 980 Hanson Group CSL 5306 Xe lamp (290 -900 nm) 250 -900 nm PMT (single wavelength) Measures lifetimes 5 ms- 1 s
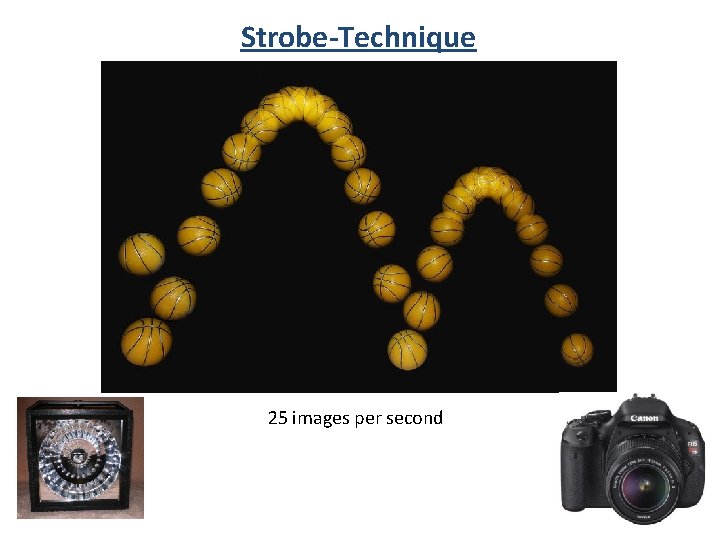
Strobe-Technique 25 images per second
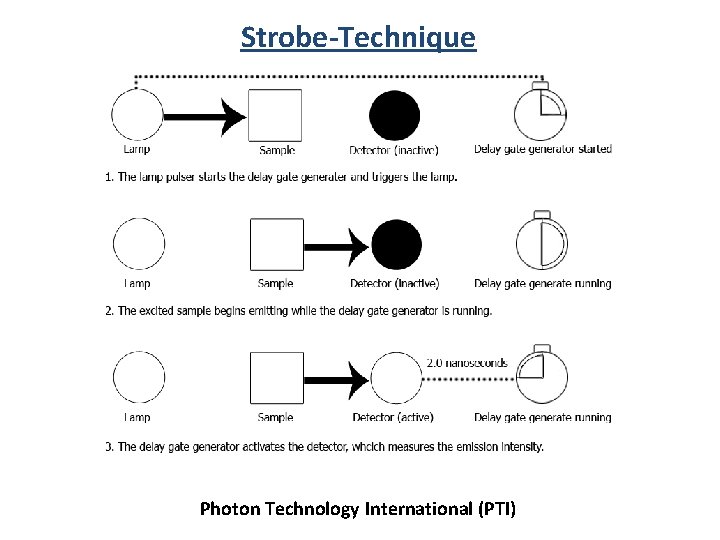
Strobe-Technique Photon Technology International (PTI)
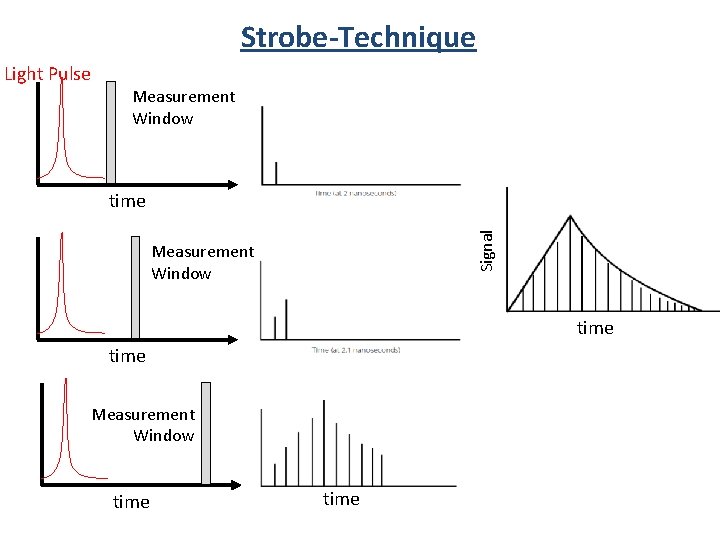
Strobe-Technique Light Pulse Measurement Window Signal time Measurement Window time
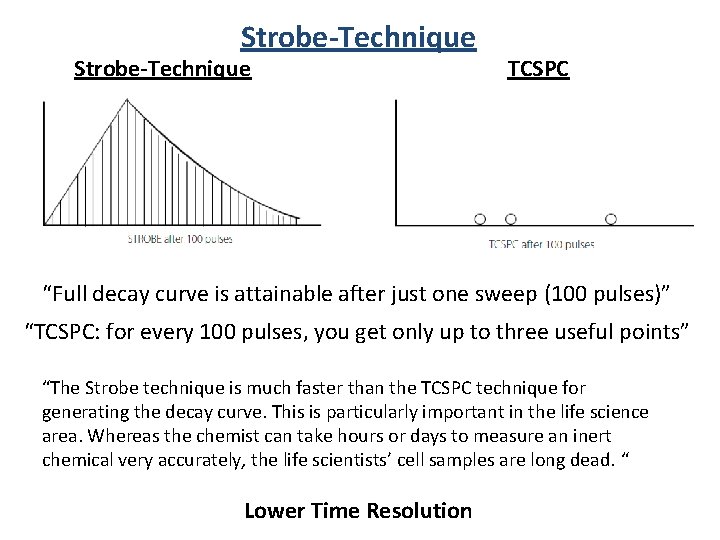
Strobe-Technique TCSPC “Full decay curve is attainable after just one sweep (100 pulses)” “TCSPC: for every 100 pulses, you get only up to three useful points” “The Strobe technique is much faster than the TCSPC technique for generating the decay curve. This is particularly important in the life science area. Whereas the chemist can take hours or days to measure an inert chemical very accurately, the life scientists’ cell samples are long dead. “ Lower Time Resolution
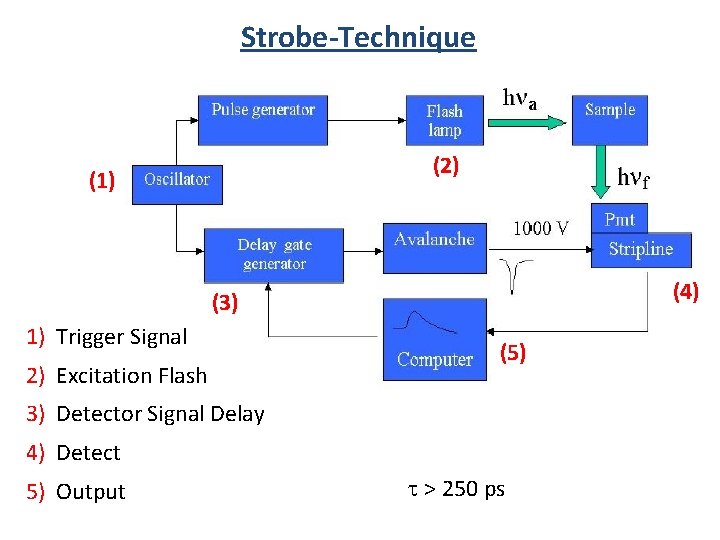
Strobe-Technique (2) (1) (4) (3) 1) Trigger Signal 2) Excitation Flash (5) 3) Detector Signal Delay 4) Detect 5) Output > 250 ps
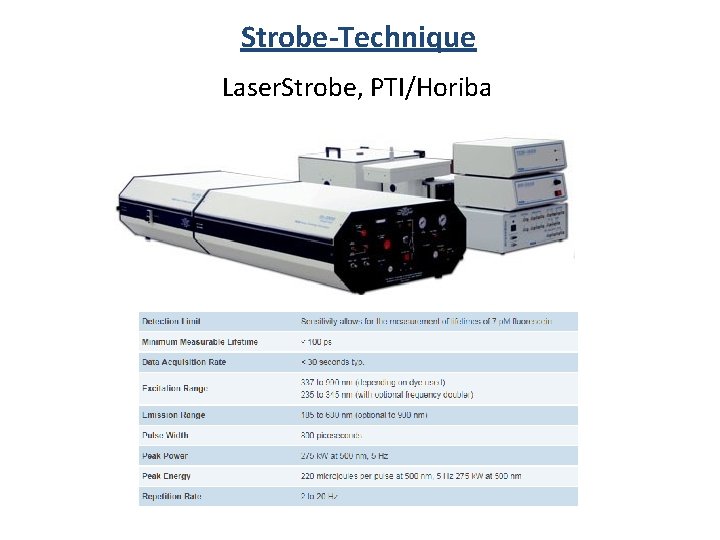
Strobe-Technique Laser. Strobe, PTI/Horiba
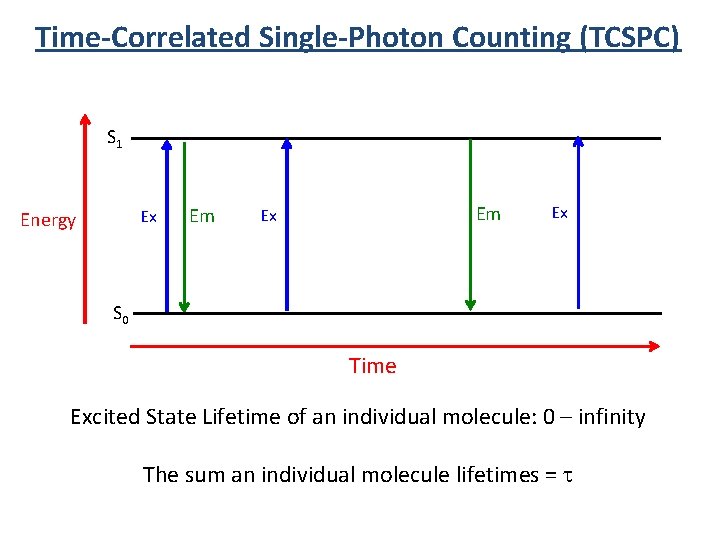
Time-Correlated Single-Photon Counting (TCSPC) S 1 Ex Energy Em Em Ex Ex S 0 Time Excited State Lifetime of an individual molecule: 0 – infinity The sum an individual molecule lifetimes =
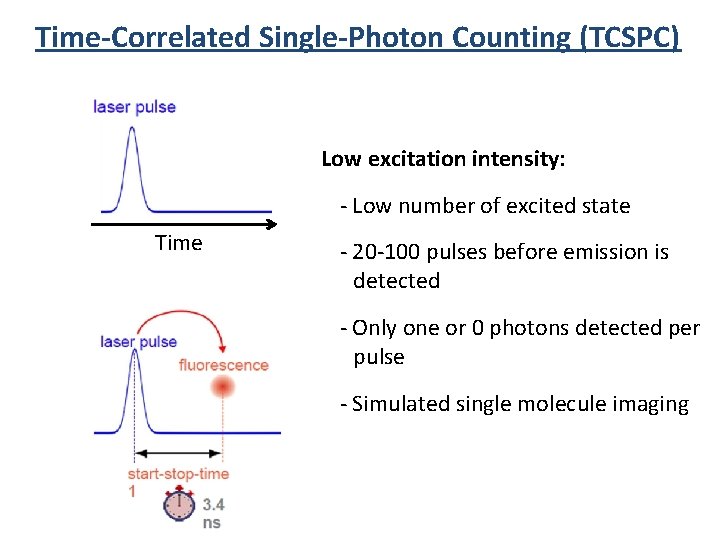
Time-Correlated Single-Photon Counting (TCSPC) Low excitation intensity: - Low number of excited state Time - 20 -100 pulses before emission is detected - Only one or 0 photons detected per pulse - Simulated single molecule imaging
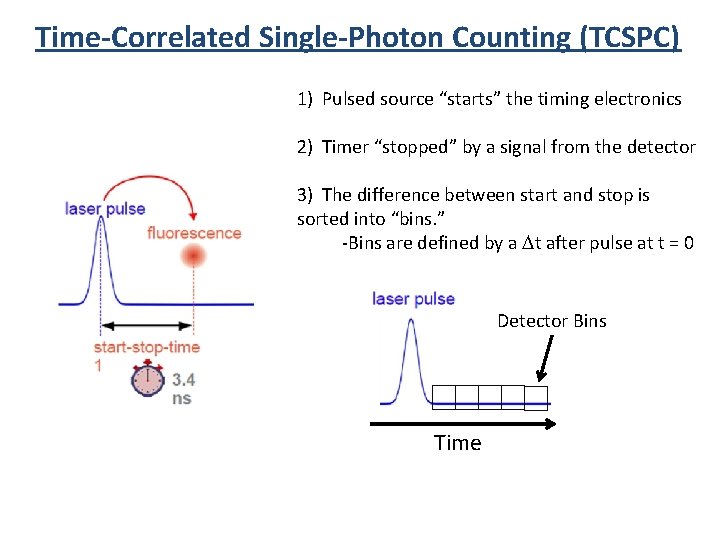
Time-Correlated Single-Photon Counting (TCSPC) 1) Pulsed source “starts” the timing electronics 2) Timer “stopped” by a signal from the detector 3) The difference between start and stop is sorted into “bins. ” -Bins are defined by a t after pulse at t = 0 Detector Bins Time
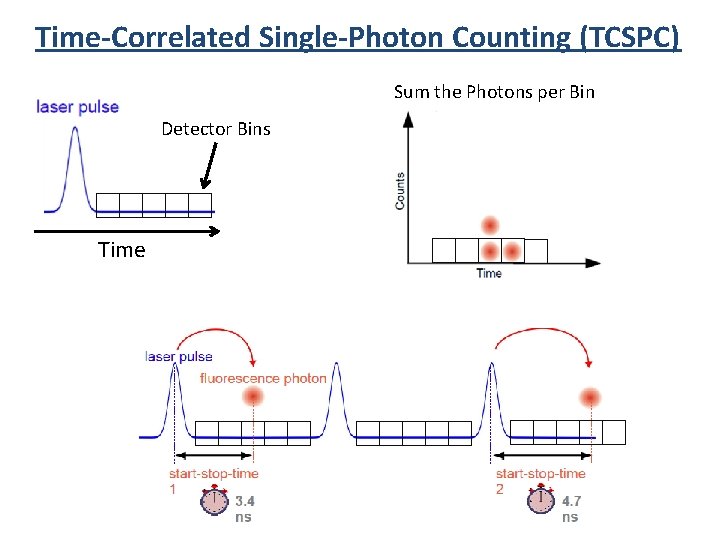
Time-Correlated Single-Photon Counting (TCSPC) Sum the Photons per Bin Detector Bins Time
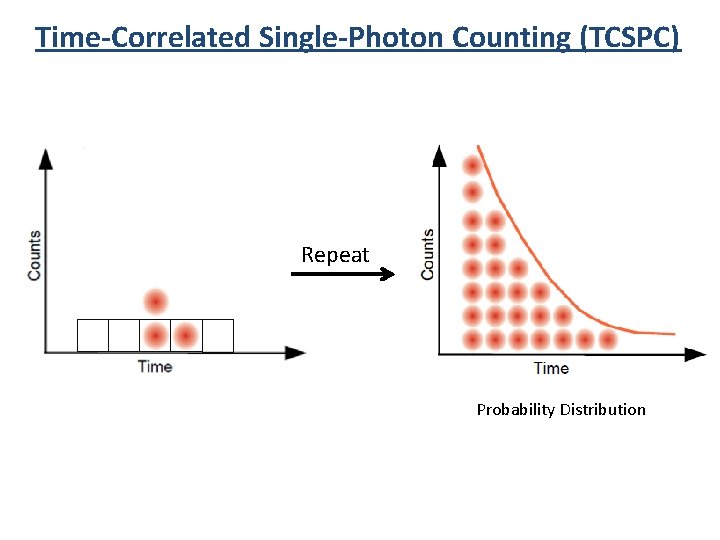
Time-Correlated Single-Photon Counting (TCSPC) Repeat Probability Distribution
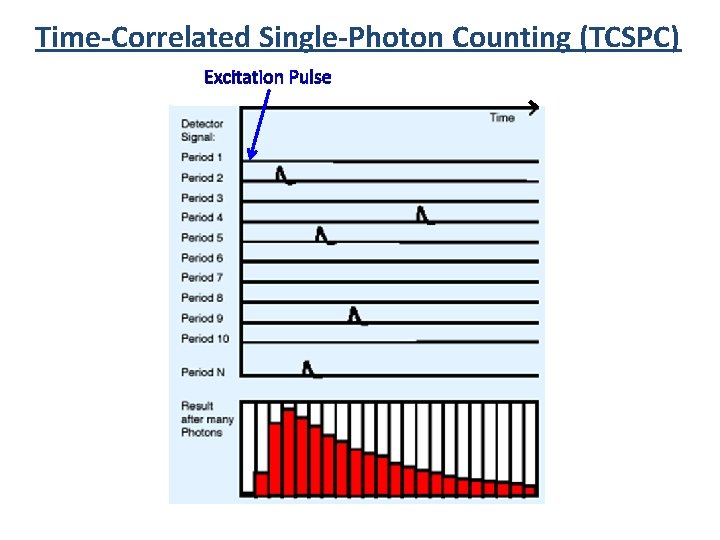
Time-Correlated Single-Photon Counting (TCSPC) Excitation Pulse
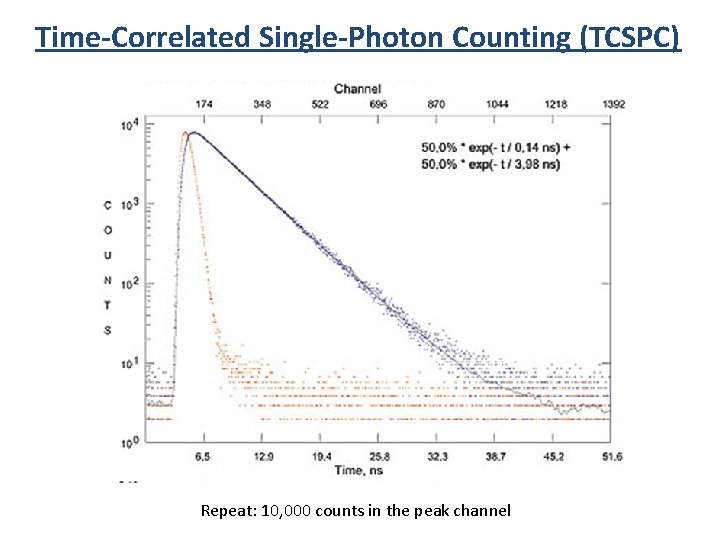
Time-Correlated Single-Photon Counting (TCSPC) Repeat: 10, 000 counts in the peak channel
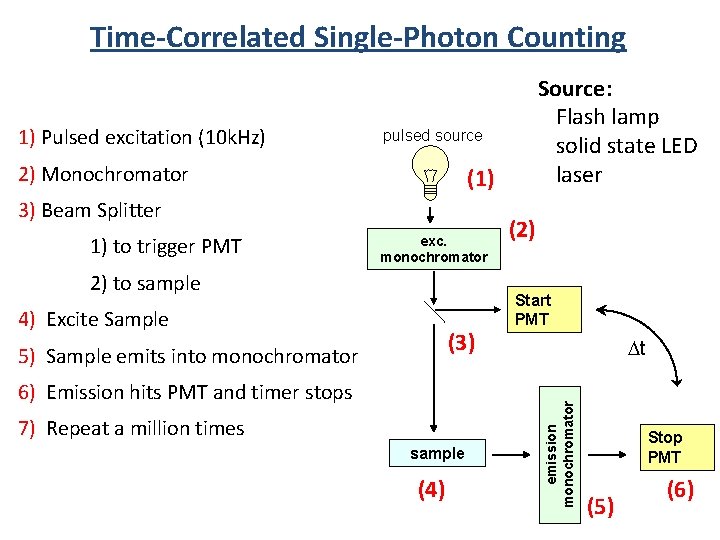
Time-Correlated Single-Photon Counting pulsed source 2) Monochromator (1) 3) Beam Splitter 1) to trigger PMT exc. monochromator 2) to sample 4) Excite Sample (3) 5) Sample emits into monochromator 6) Emission hits PMT and timer stops 7) Repeat a million times sample (4) (2) Start PMT t emission monochromator 1) Pulsed excitation (10 k. Hz) Source: Flash lamp solid state LED laser Stop PMT (5) (6)
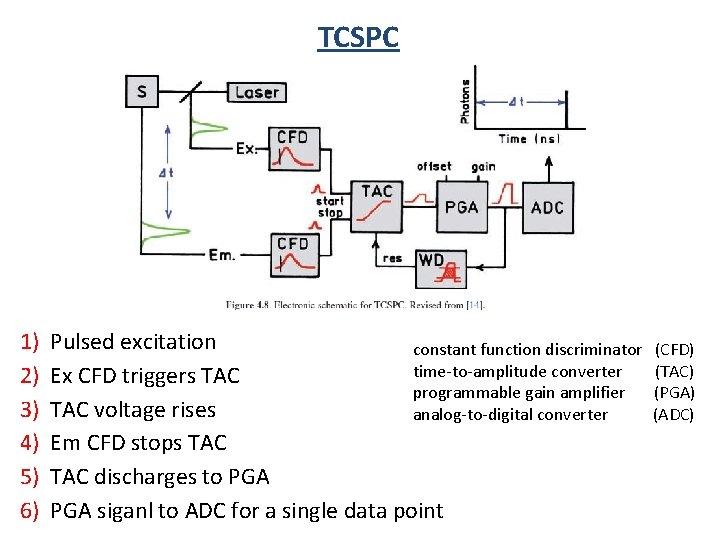
TCSPC 1) Pulsed excitation constant function discriminator (CFD) time-to-amplitude converter (TAC) 2) Ex CFD triggers TAC programmable gain amplifier (PGA) 3) TAC voltage rises analog-to-digital converter (ADC) 4) Em CFD stops TAC 5) TAC discharges to PGA 6) PGA siganl to ADC for a single data point
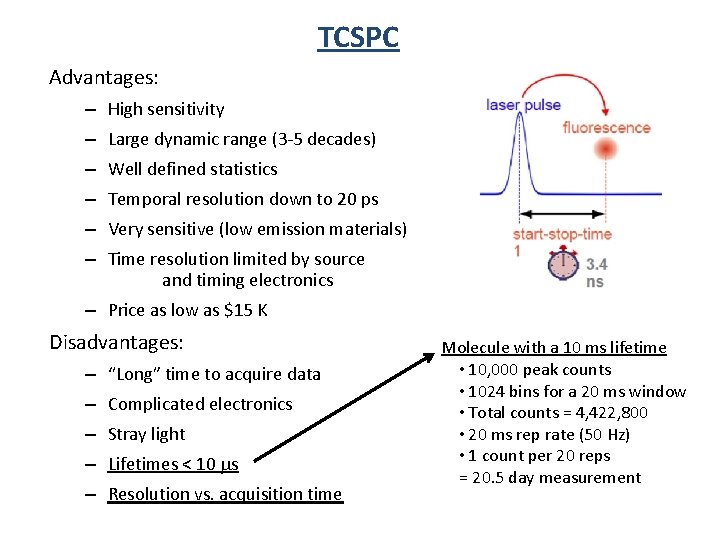
TCSPC Advantages: – High sensitivity – Large dynamic range (3 -5 decades) – Well defined statistics – Temporal resolution down to 20 ps – Very sensitive (low emission materials) – Time resolution limited by source and timing electronics – Price as low as $15 K Disadvantages: – “Long” time to acquire data – Complicated electronics – Stray light – Lifetimes < 10 ms – Resolution vs. acquisition time Molecule with a 10 ms lifetime • 10, 000 peak counts • 1024 bins for a 20 ms window • Total counts = 4, 422, 800 • 20 ms rep rate (50 Hz) • 1 count per 20 reps = 20. 5 day measurement
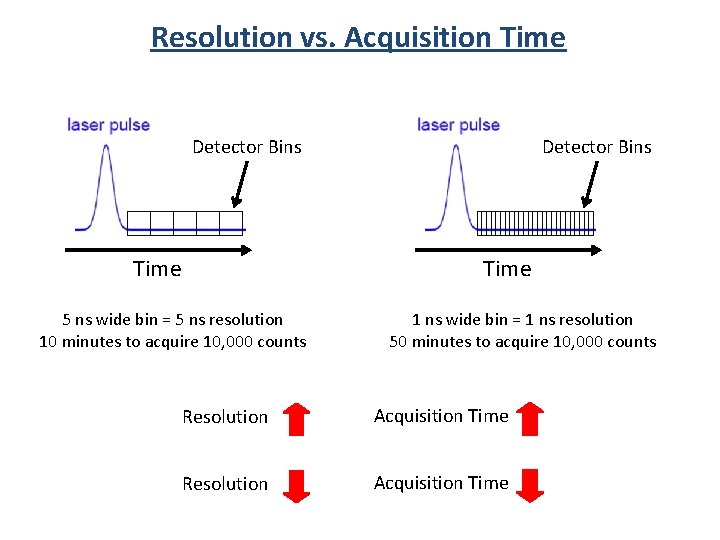
Resolution vs. Acquisition Time Detector Bins Time 5 ns wide bin = 5 ns resolution 10 minutes to acquire 10, 000 counts 1 ns wide bin = 1 ns resolution 50 minutes to acquire 10, 000 counts Resolution Acquisition Time
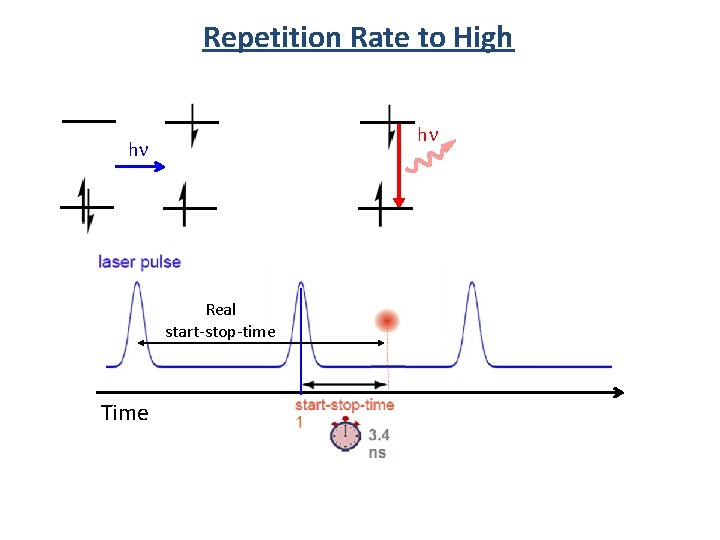
Repetition Rate to High hn hn Real start-stop-time Time
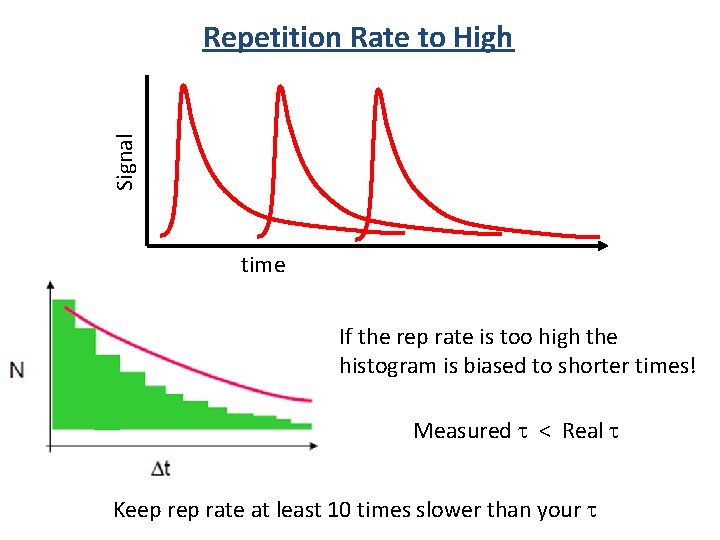
Signal Repetition Rate to High time If the rep rate is too high the histogram is biased to shorter times! Measured < Real Keep rate at least 10 times slower than your
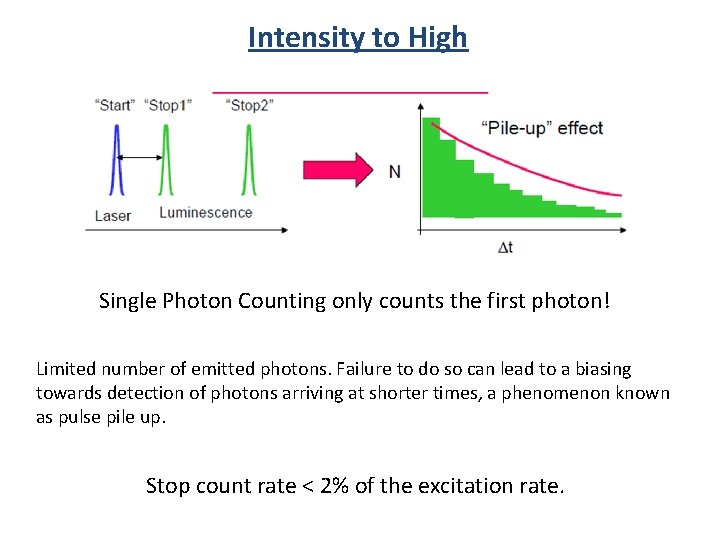
Intensity to High Single Photon Counting only counts the first photon! Limited number of emitted photons. Failure to do so can lead to a biasing towards detection of photons arriving at shorter times, a phenomenon known as pulse pile up. Stop count rate < 2% of the excitation rate.
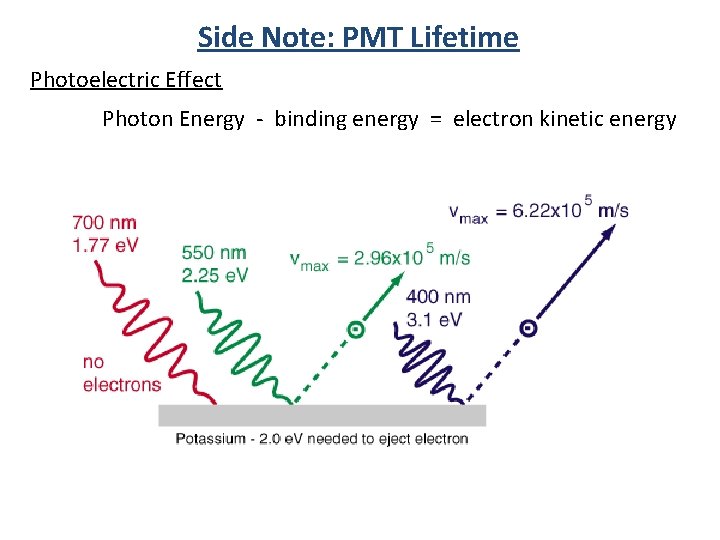
Side Note: PMT Lifetime Photoelectric Effect Photon Energy - binding energy = electron kinetic energy
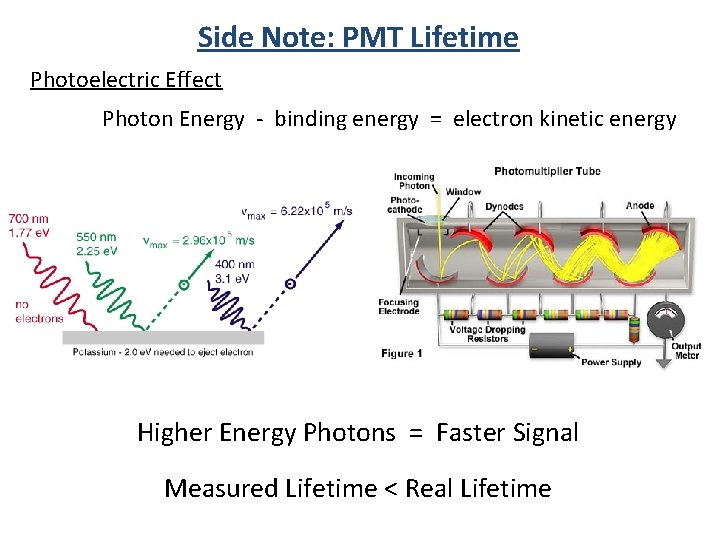
Side Note: PMT Lifetime Photoelectric Effect Photon Energy - binding energy = electron kinetic energy Higher Energy Photons = Faster Signal Measured Lifetime < Real Lifetime
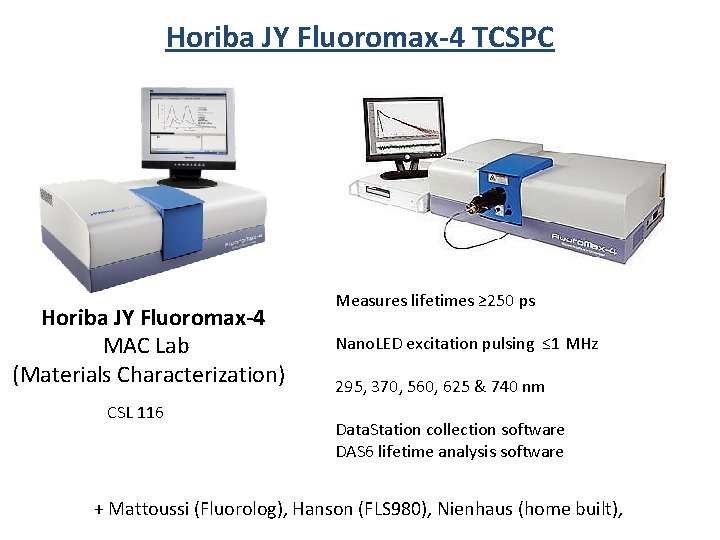
Horiba JY Fluoromax-4 TCSPC Horiba JY Fluoromax-4 MAC Lab (Materials Characterization) CSL 116 Measures lifetimes ≥ 250 ps Nano. LED excitation pulsing ≤ 1 MHz 295, 370, 560, 625 & 740 nm Data. Station collection software DAS 6 lifetime analysis software + Mattoussi (Fluorolog), Hanson (FLS 980), Nienhaus (home built),
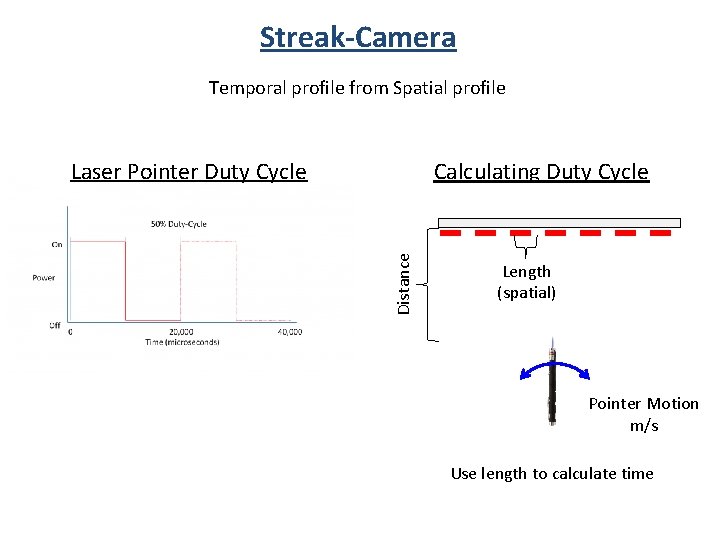
Streak-Camera Temporal profile from Spatial profile Laser Pointer Duty Cycle Distance Calculating Duty Cycle Length (spatial) Pointer Motion m/s Use length to calculate time
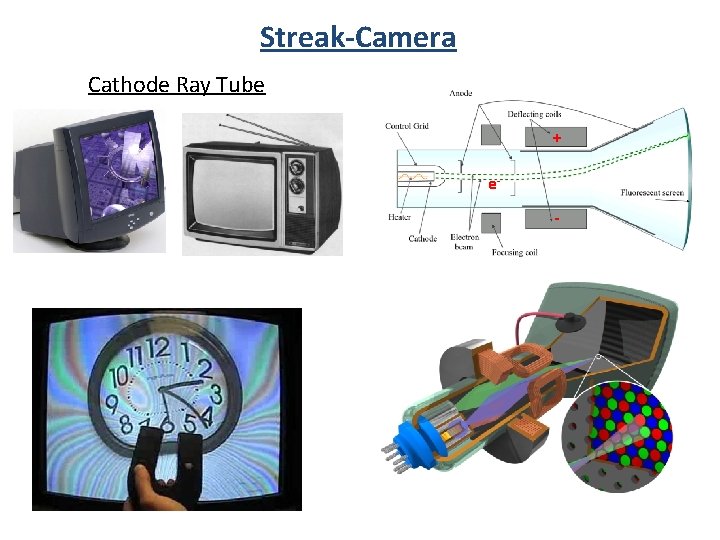
Streak-Camera Cathode Ray Tube + e-
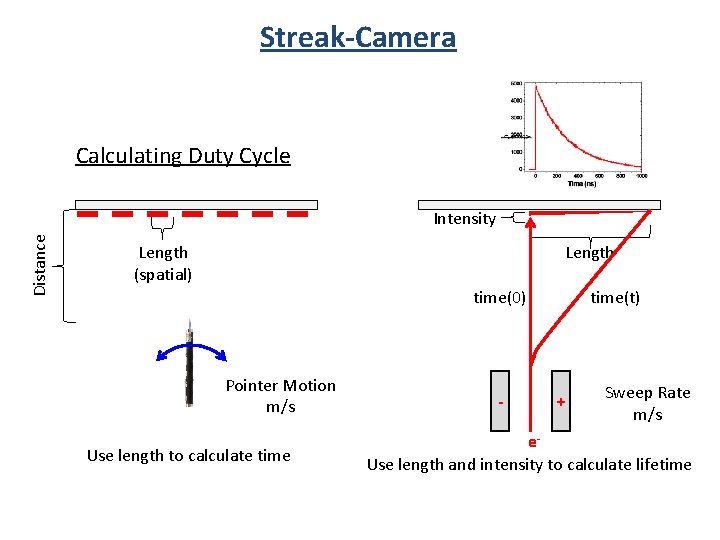
Streak-Camera Calculating Duty Cycle Distance Intensity Length (spatial) Length time(0) Pointer Motion m/s Use length to calculate time - time(t) + Sweep Rate m/s e. Use length and intensity to calculate lifetime
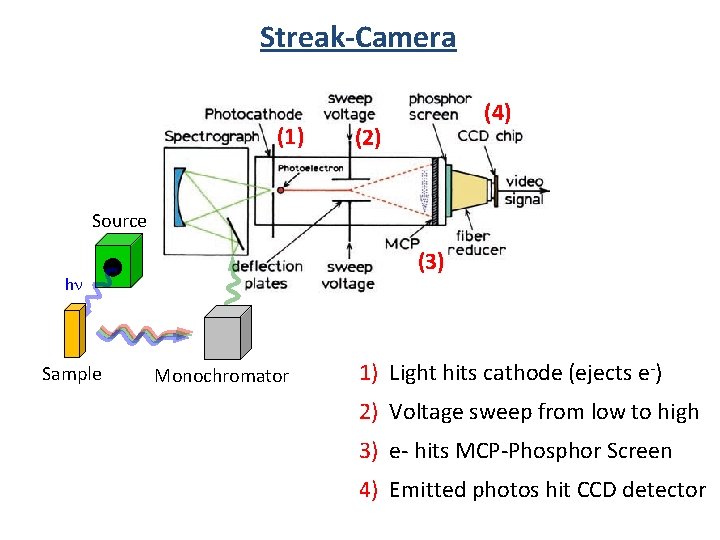
Streak-Camera (1) (4) (2) Source (3) hn Sample Monochromator 1) Light hits cathode (ejects e-) 2) Voltage sweep from low to high 3) e- hits MCP-Phosphor Screen 4) Emitted photos hit CCD detector
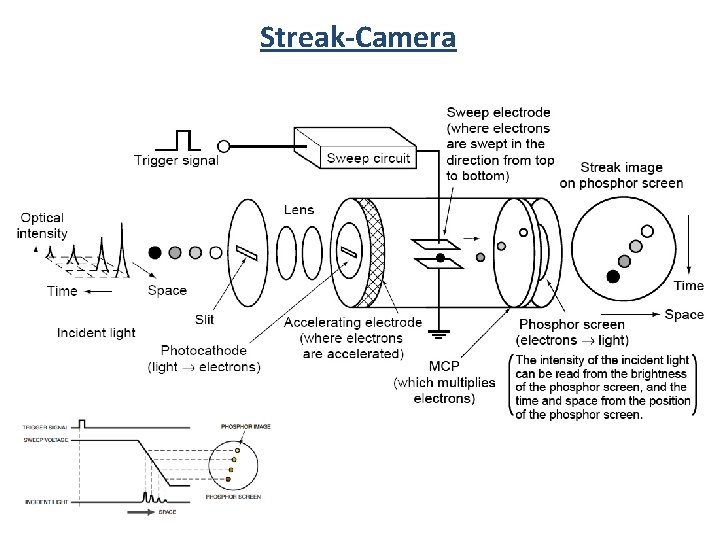
Streak-Camera
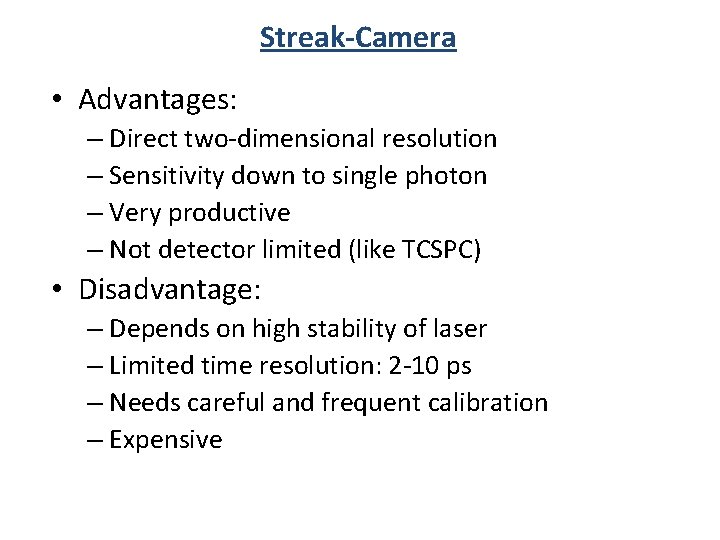
Streak-Camera • Advantages: – Direct two-dimensional resolution – Sensitivity down to single photon – Very productive – Not detector limited (like TCSPC) • Disadvantage: – Depends on high stability of laser – Limited time resolution: 2 -10 ps – Needs careful and frequent calibration – Expensive
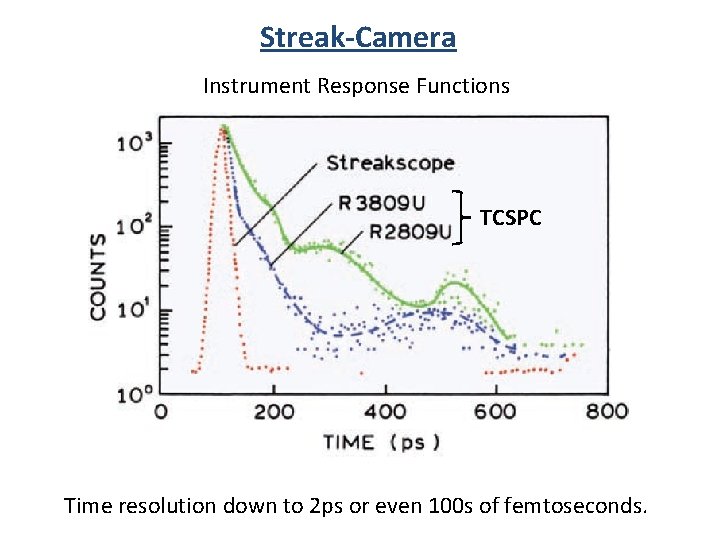
Streak-Camera Instrument Response Functions TCSPC Time resolution down to 2 ps or even 100 s of femtoseconds.
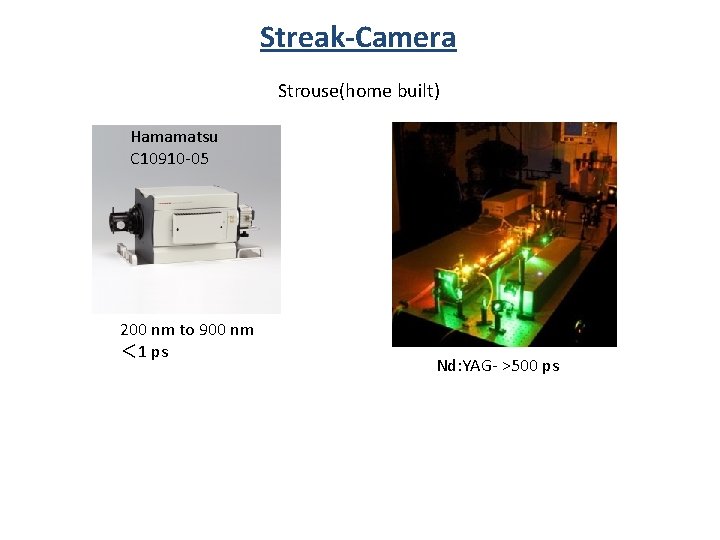
Streak-Camera Strouse(home built) Hamamatsu C 10910 -05 200 nm to 900 nm < 1 ps Nd: YAG- >500 ps
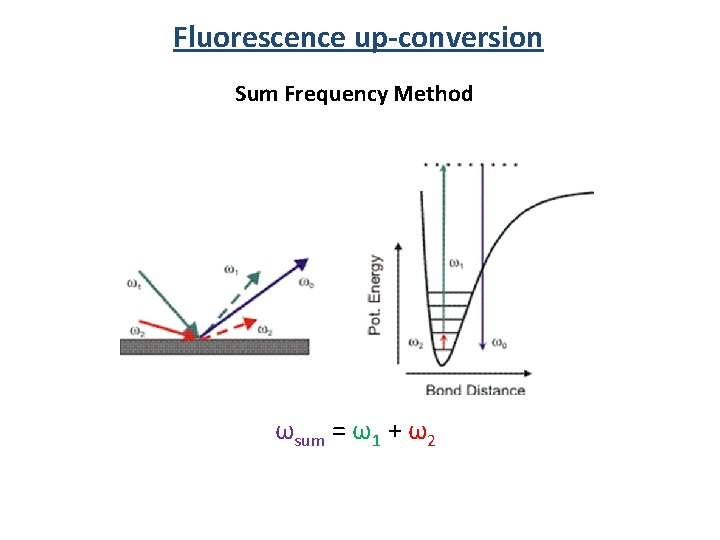
Fluorescence up-conversion Sum Frequency Method ωsum = ω1 + ω2
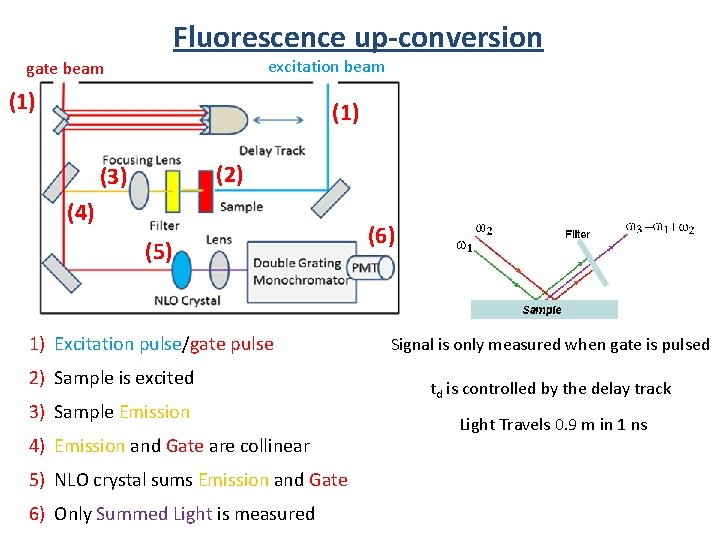
Fluorescence up-conversion excitation beam gate beam (1) (2) (3) (4) (5) 1) Excitation pulse/gate pulse 2) Sample is excited 3) Sample Emission 4) Emission and Gate are collinear 5) NLO crystal sums Emission and Gate 6) Only Summed Light is measured (6) Signal is only measured when gate is pulsed td is controlled by the delay track Light Travels 0. 9 m in 1 ns
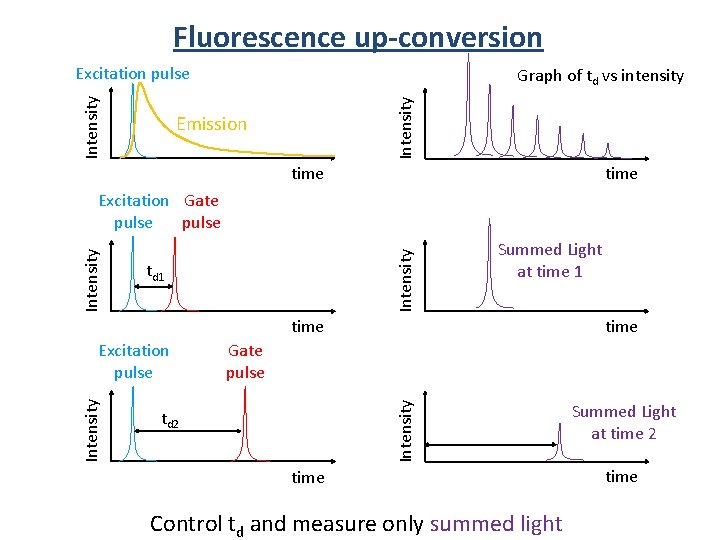
Fluorescence up-conversion Graph of td vs intensity Intensity Excitation pulse Emission time Intensity Excitation Gate pulse td 1 Summed Light at time 1 time Gate pulse Intensity Excitation pulse td 2 time Control td and measure only summed light Summed Light at time 2 time
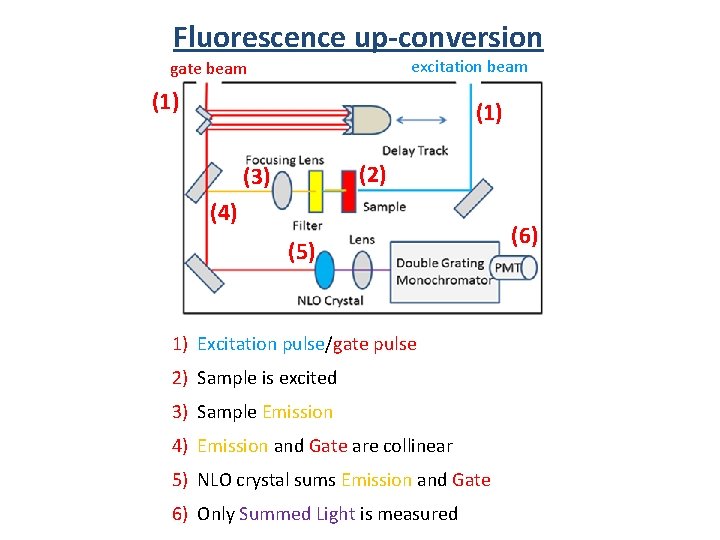
Fluorescence up-conversion excitation beam gate beam (1) (2) (3) (4) (5) 1) Excitation pulse/gate pulse 2) Sample is excited 3) Sample Emission 4) Emission and Gate are collinear 5) NLO crystal sums Emission and Gate 6) Only Summed Light is measured (6)
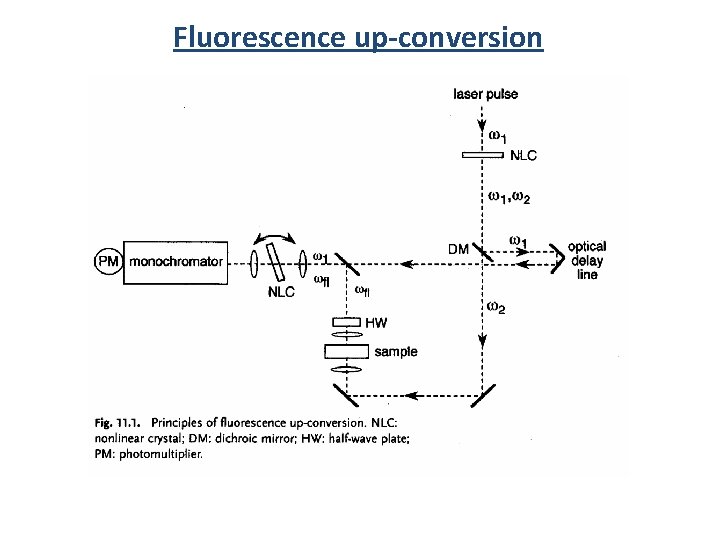
Fluorescence up-conversion
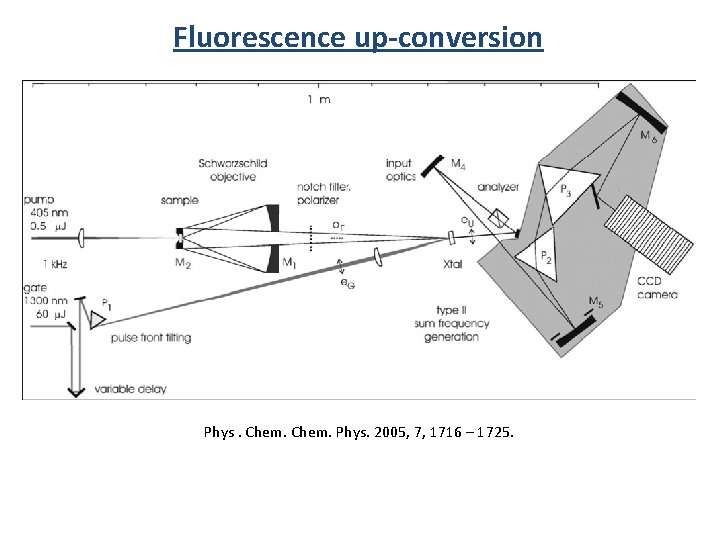
Fluorescence up-conversion Phys. Chem. Phys. 2005, 7, 1716 – 1725.
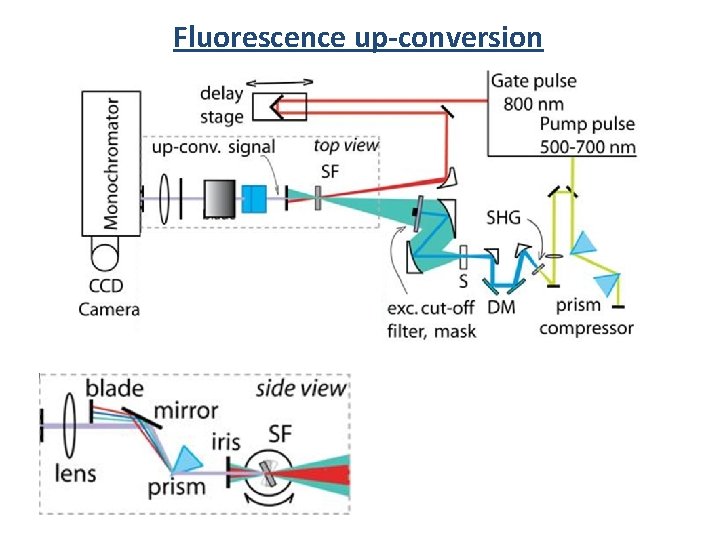
Fluorescence up-conversion
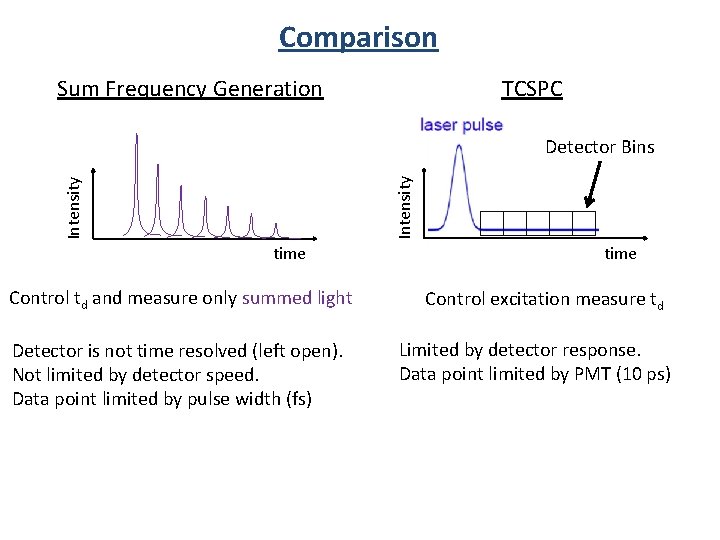
Comparison Sum Frequency Generation TCSPC Intensity Detector Bins time Control td and measure only summed light Detector is not time resolved (left open). Not limited by detector speed. Data point limited by pulse width (fs) time Control excitation measure td Limited by detector response. Data point limited by PMT (10 ps)
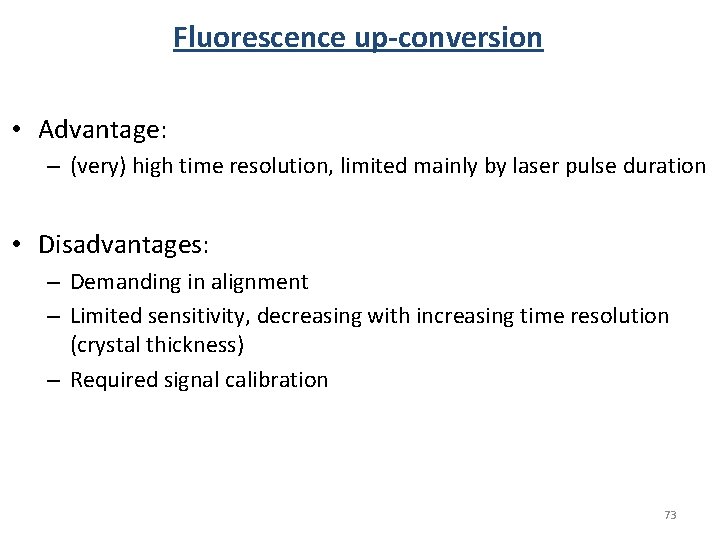
Fluorescence up-conversion • Advantage: – (very) high time resolution, limited mainly by laser pulse duration • Disadvantages: – Demanding in alignment – Limited sensitivity, decreasing with increasing time resolution (crystal thickness) – Required signal calibration 73
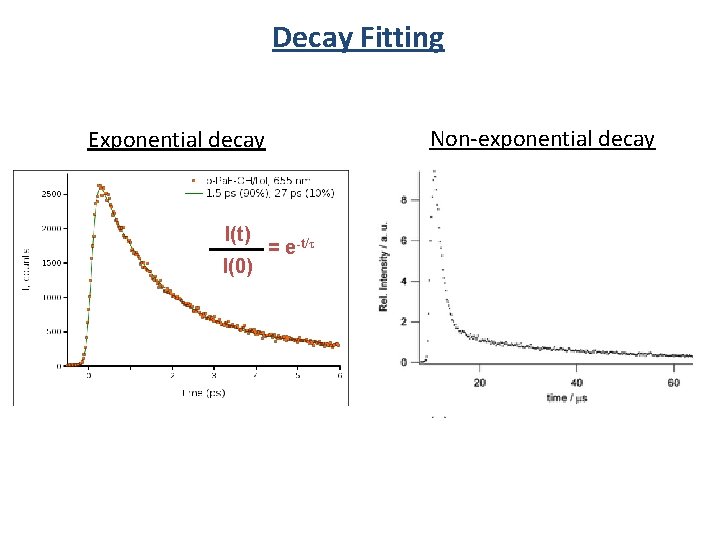
Decay Fitting Non-exponential decay Exponential decay I(t) I(0) = e-t/
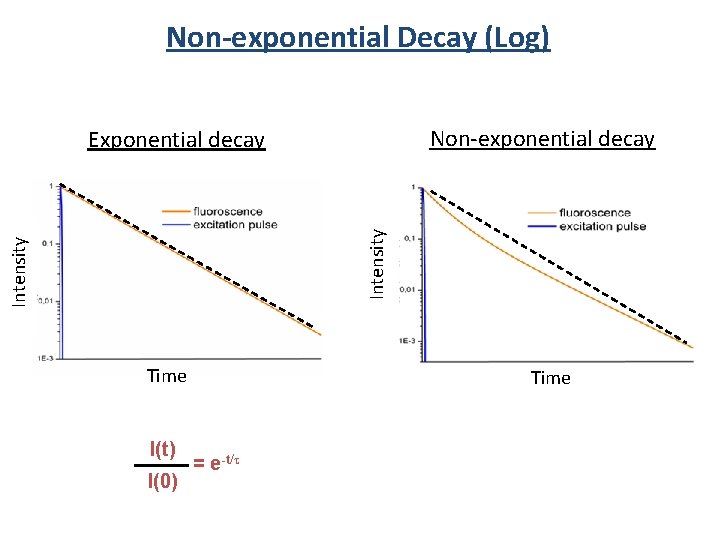
Non-exponential Decay (Log) Non-exponential decay Intensity Exponential decay Time I(t) I(0) Time = e-t/
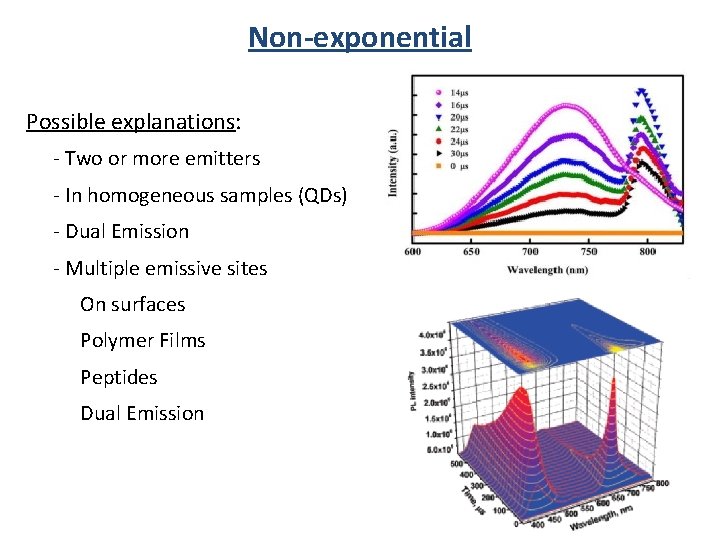
Non-exponential Possible explanations: - Two or more emitters - In homogeneous samples (QDs) - Dual Emission - Multiple emissive sites On surfaces Polymer Films Peptides Dual Emission
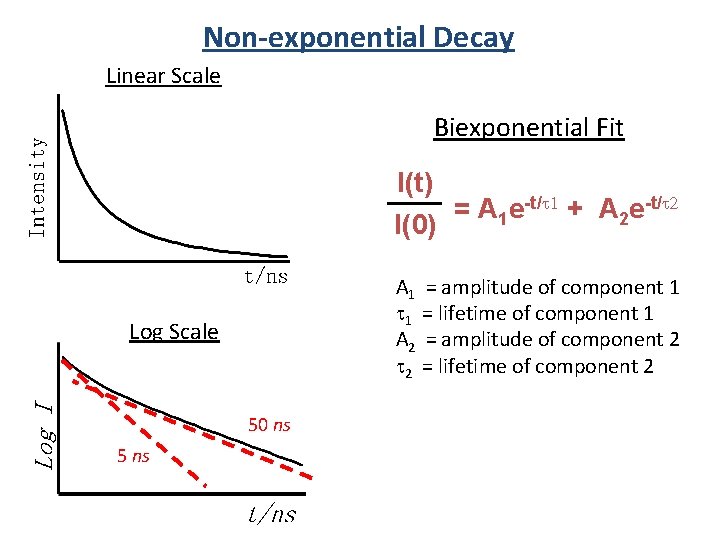
Non-exponential Decay Linear Scale Biexponential Fit I(t) = A 1 e-t/ 1 + A 2 e-t/ 2 I(0) A 1 = amplitude of component 1 1 = lifetime of component 1 A 2 = amplitude of component 2 2 = lifetime of component 2 Log Scale 50 ns 5 ns
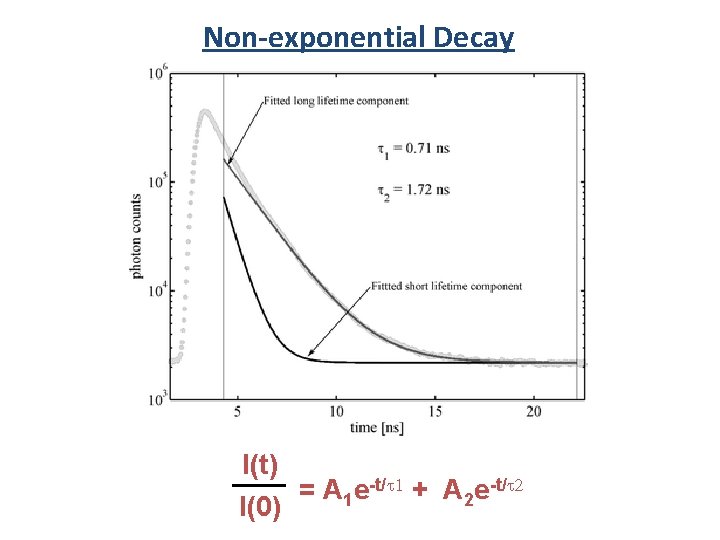
Non-exponential Decay I(t) = A 1 e-t/ 1 + A 2 e-t/ 2 I(0)
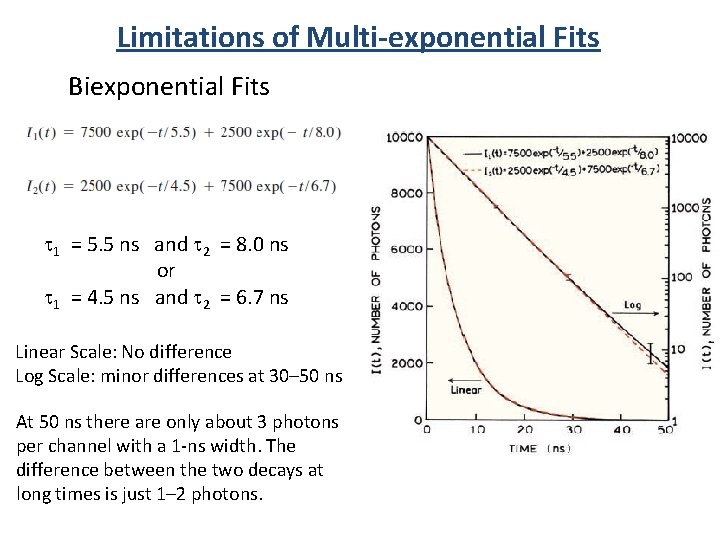
Limitations of Multi-exponential Fits Biexponential Fits 1 = 5. 5 ns and 2 = 8. 0 ns or 1 = 4. 5 ns and 2 = 6. 7 ns Linear Scale: No difference Log Scale: minor differences at 30– 50 ns At 50 ns there are only about 3 photons per channel with a 1 -ns width. The difference between the two decays at long times is just 1– 2 photons.
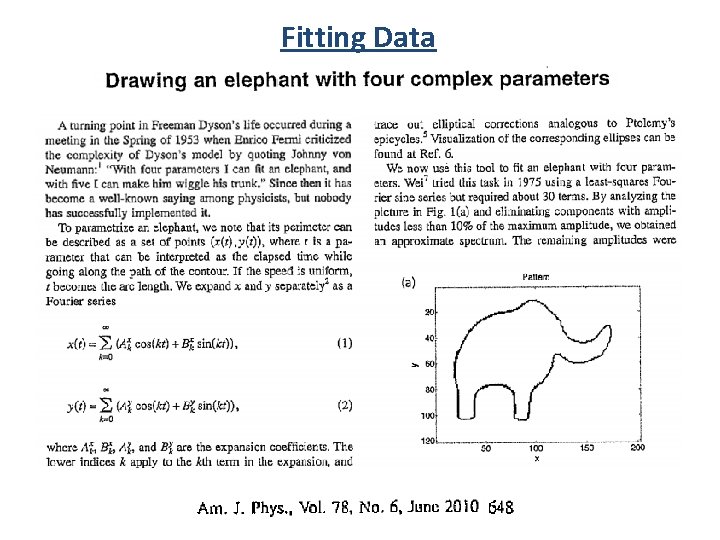
Fitting Data
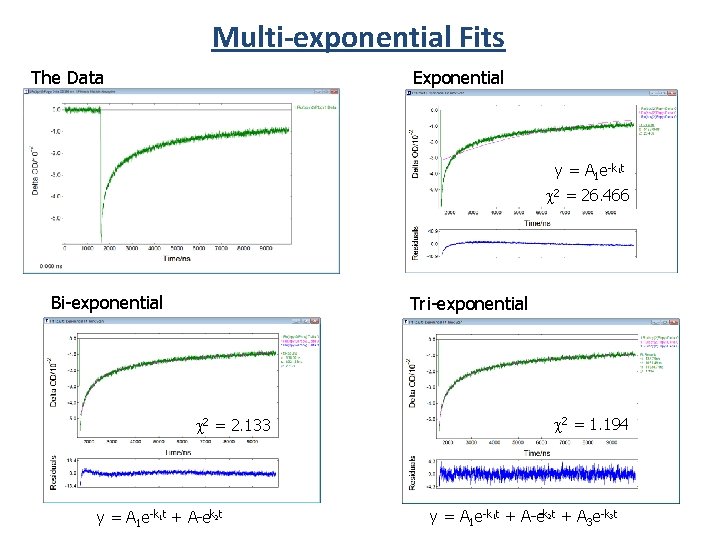
Multi-exponential Fits Exponential The Data y = A 1 e k 1 t c 2 = 26. 466 Bi exponential Tri exponential c 2 = 2. 133 y = A 1 e k 1 t + A e k 2 t c 2 = 1. 194 y = A 1 e k 1 t + A e k 2 t + A 3 e k 3 t
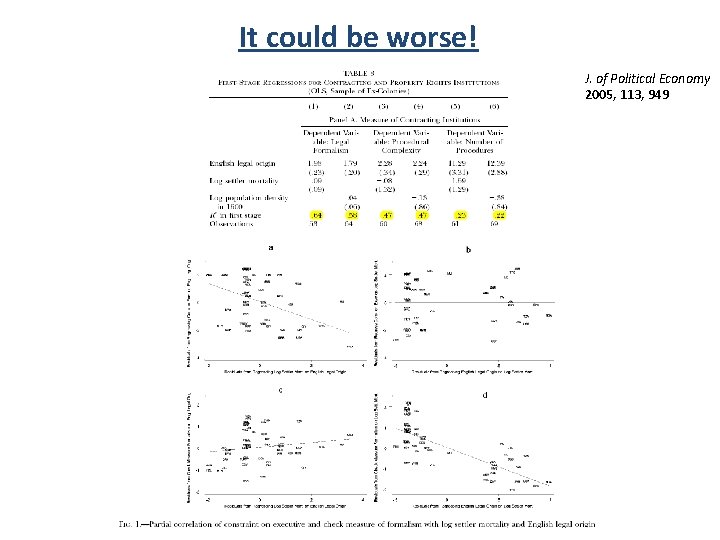
It could be worse! J. of Political Economy 2005, 113, 949
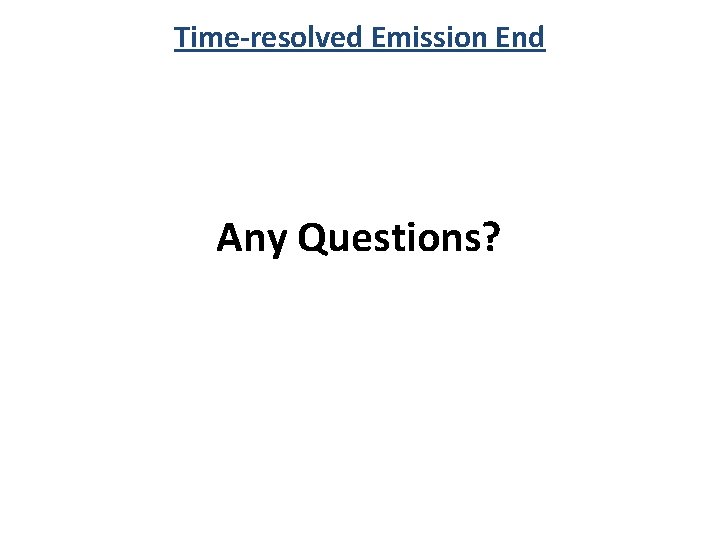
Time-resolved Emission End Any Questions?
Fast clock to slow clock synchronization
60 seconds per minute times 60 minutes per hour
Chm 151 final exam
Chemistry
Maskants and etchants
Chem 130 final exam
Chm 241
Chm 201
Chm 1045
Chm 111
Chm machining
Chm tool
Chm logo
Cobalt-60 nuclear decay equation
What is the symbol for an alpha particle
Positron emission
Einstein equation for photoelectric emission is
Maser vs laser
Metastable state
Population inversion laser
Spontaneous emission
Bandplan tingkat siaga pada band vhf
Bread toasting: gas formation color change light emission
Beta minus decay
Researchgate
Principle of flame test
Alpha particle in electric field
Atomic emission spectroscopy ppt
Beilstein test
Atomic emission spectroscopy principle
Difference between absorption and emission spectrum
Emission vs ejaculation
Miller zoo emission
Emission line spectrum
Atomic emission spectra and the quantum mechanical model
Neutrino lepton number
Is code 800
Photo emission microscopy failure analysis
Plastic oled
Atomic emission spectra periodic table
Cispr 22 radiated emission limits
Flame photometry instrumentation
Emission vs ejaculation
Alpha decay
Acoustic emissions testing
Atomic emission spectra and the quantum mechanical model
Positron emission tomography
Positron emission tomography
Complexe g emission
Matter and materials grade 12
Why are atomic emission spectra discontinuous
Atomic emission spectroscopy lecture notes
Energy emission
Energy emission
Pet/ct
The pcv system controls which exhaust emission(s)?
Tga vean
Transition and emission probability
Energy emission
Heisenberg uncertainty principle
Automotive technology chapter 51 answers
Emission
Carbon emission
Emission control system repair
Emission line
Absorption spectrum
Thermionic emission current
Zero emission neighbourhoods
Positron emission tomography
Positron emission tomography
Alpha emission
Half-life formula
Ipcc emission factor database
Neutron emission
Charge of electron
Hallwachs observation on photoelectric effect
Ultra low emission burner
Alfa beta gamma strålning
Sample source
Merits and demerits of cluster sampling
Volunteer sample vs convenience sample
Volunteer sample vs convenience sample
What is stratified sampling
Stratified sampling vs cluster sampling