COORDINATION CHEMISTRY COORDINATION COMPLEX Any ion or neutral
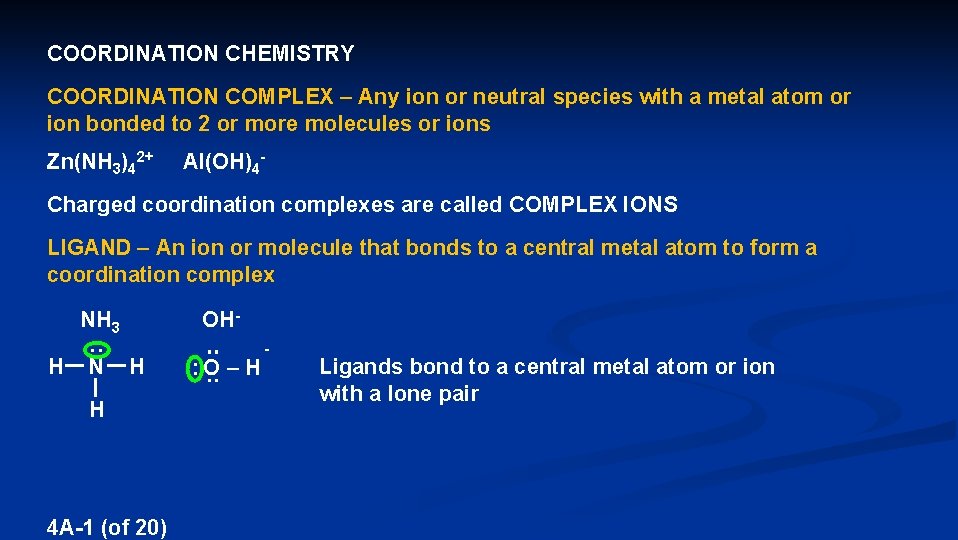
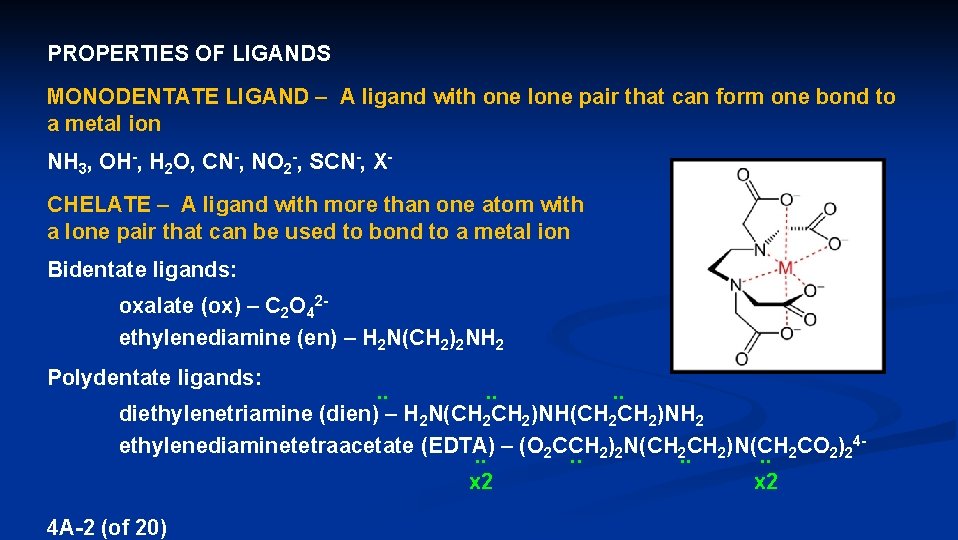
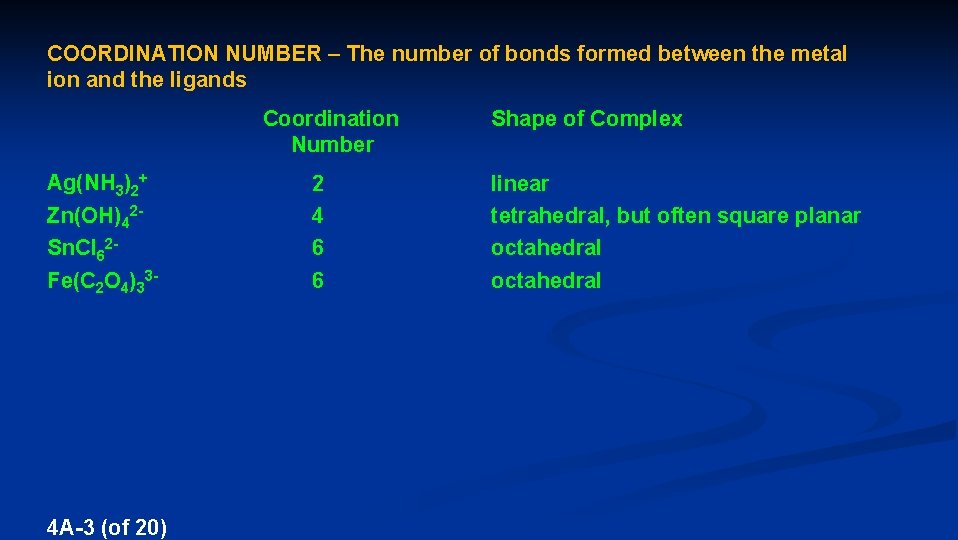
![COORDINATION COMPOUND – Any compound containing a complex ion and a counterion [Cu(NH 3)4]Cl COORDINATION COMPOUND – Any compound containing a complex ion and a counterion [Cu(NH 3)4]Cl](https://slidetodoc.com/presentation_image_h/20ac91025ecf151ea91f5edf1dffa241/image-4.jpg)
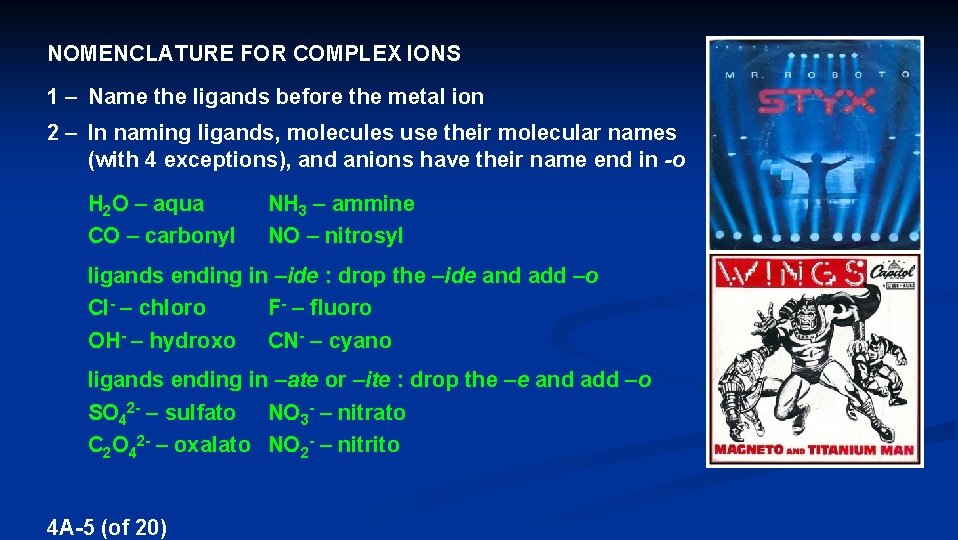
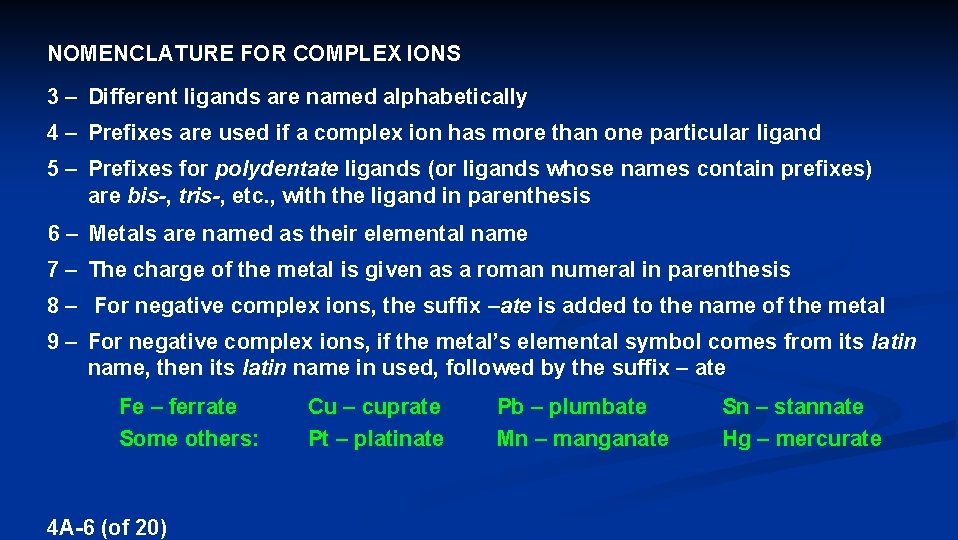
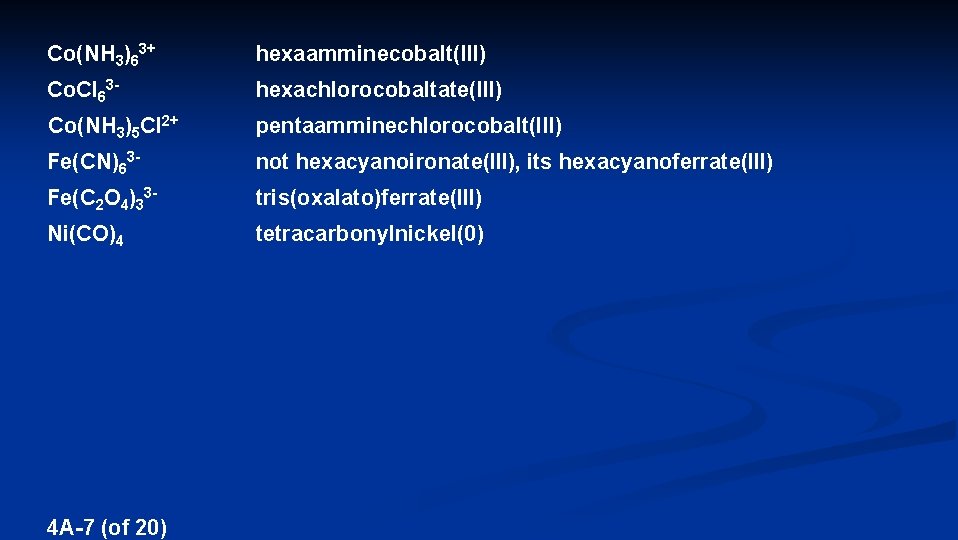
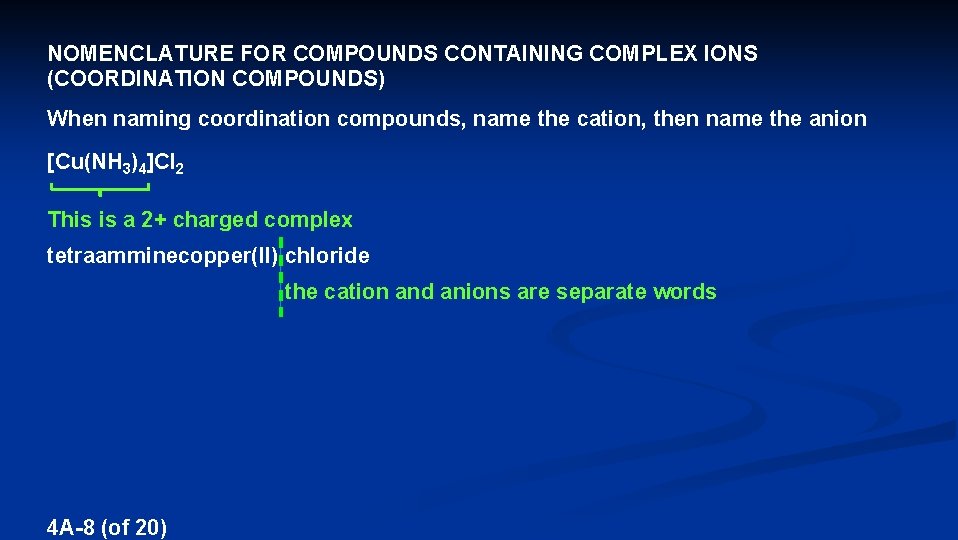
![[Cr(NH 3)6]Cl 3 This is a 3+ charged complex ion hexaamminechromium(III) chloride [Pt(NH 3)3 [Cr(NH 3)6]Cl 3 This is a 3+ charged complex ion hexaamminechromium(III) chloride [Pt(NH 3)3](https://slidetodoc.com/presentation_image_h/20ac91025ecf151ea91f5edf1dffa241/image-9.jpg)
![K[Pt. NH 3 Cl 5] This is a 1 - charged complex ion potassium K[Pt. NH 3 Cl 5] This is a 1 - charged complex ion potassium](https://slidetodoc.com/presentation_image_h/20ac91025ecf151ea91f5edf1dffa241/image-10.jpg)
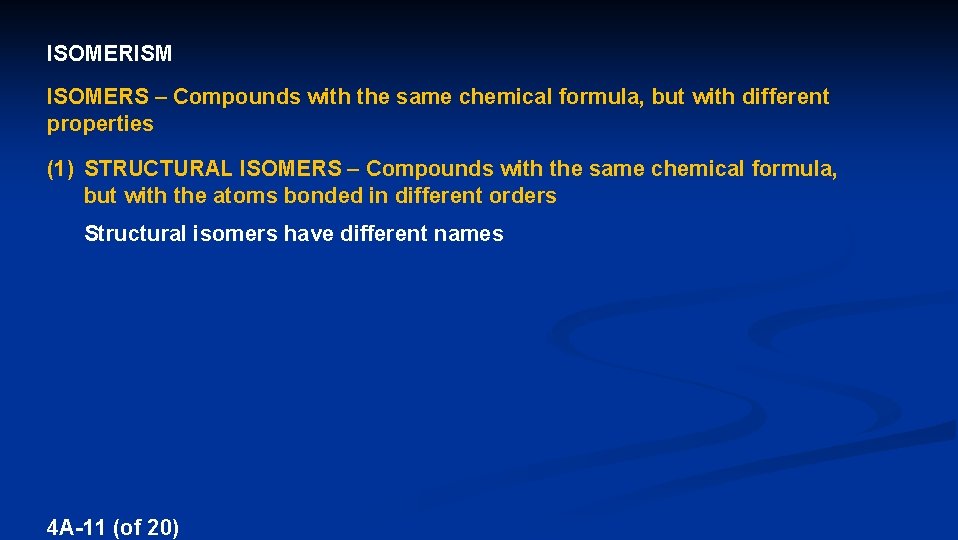
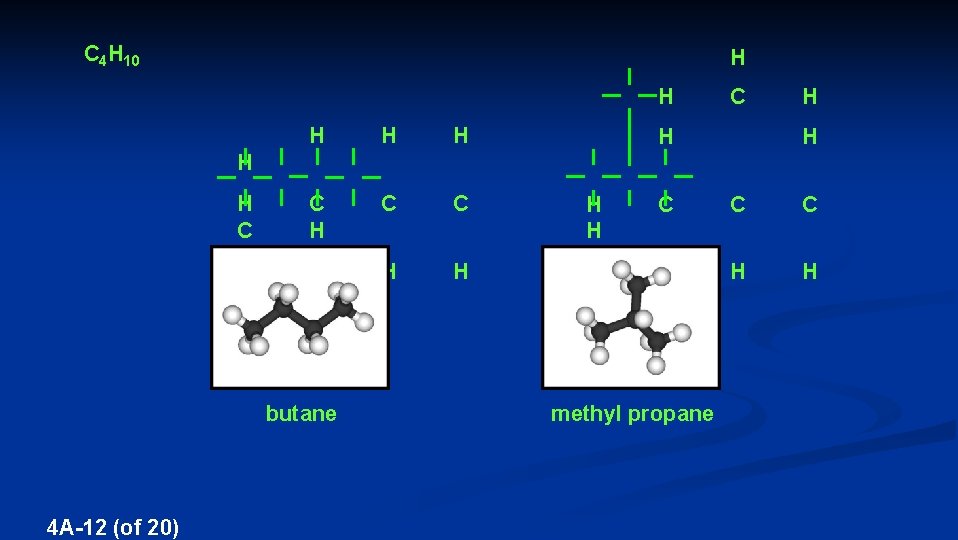
![Pt(H 2 O)4(OH)2 Cl 2 [Pt(H 2 O)4(OH)2]Cl 2 H 2 O Pt 2+ Pt(H 2 O)4(OH)2 Cl 2 [Pt(H 2 O)4(OH)2]Cl 2 H 2 O Pt 2+](https://slidetodoc.com/presentation_image_h/20ac91025ecf151ea91f5edf1dffa241/image-13.jpg)
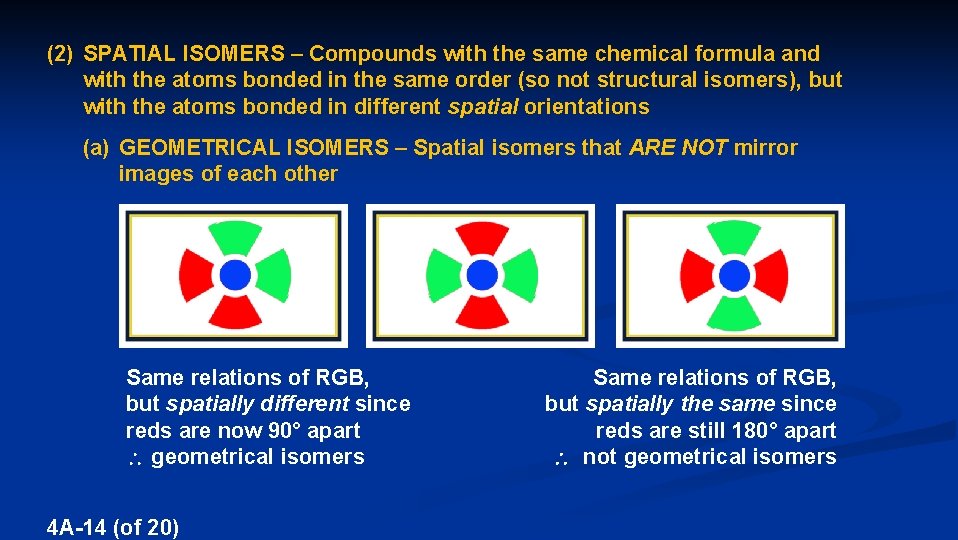
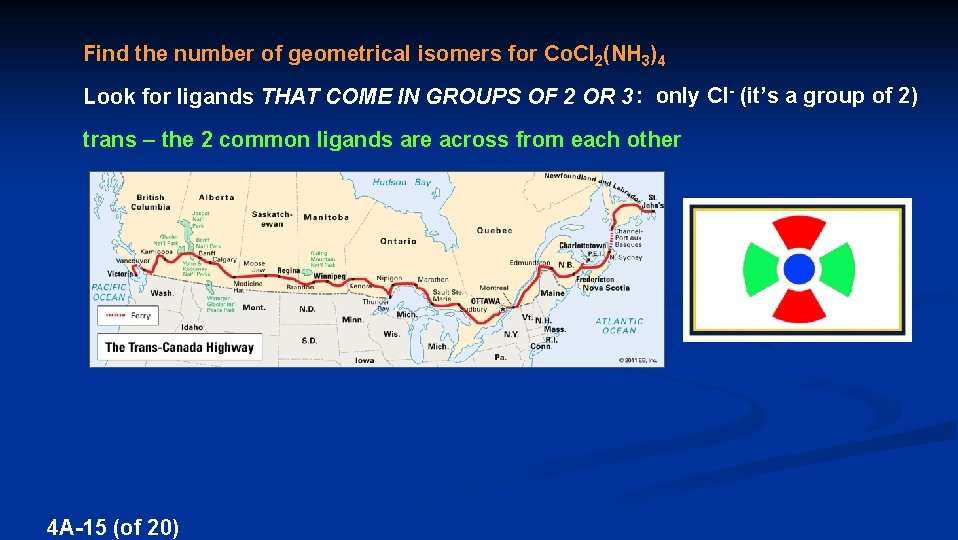
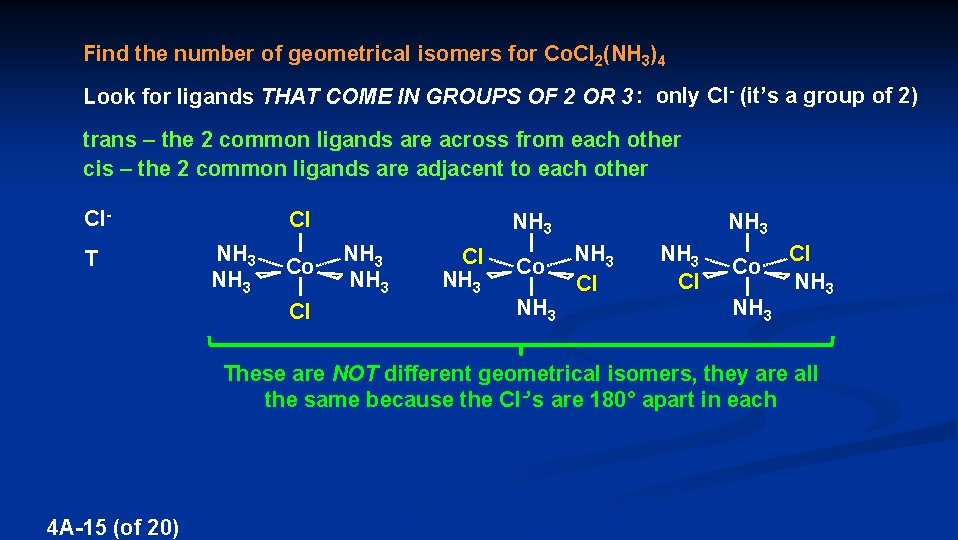
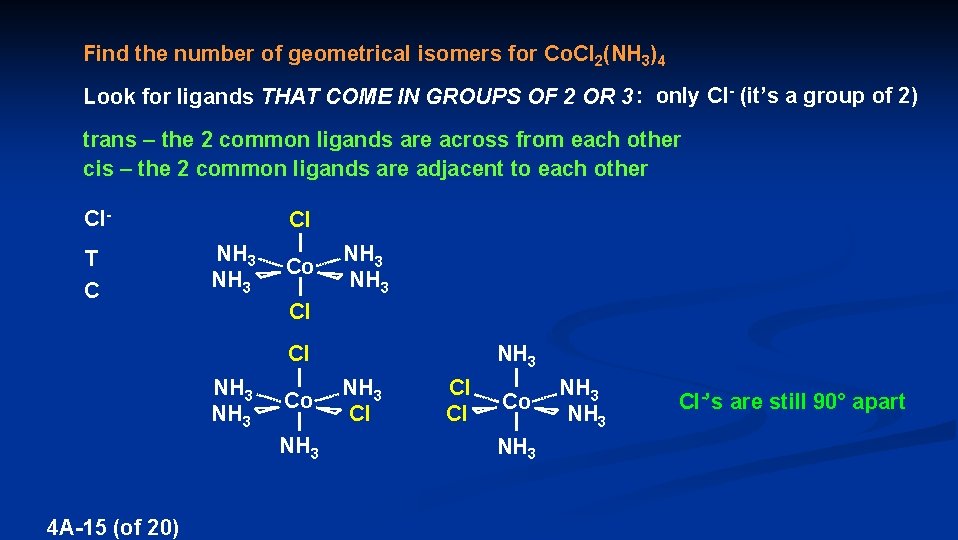
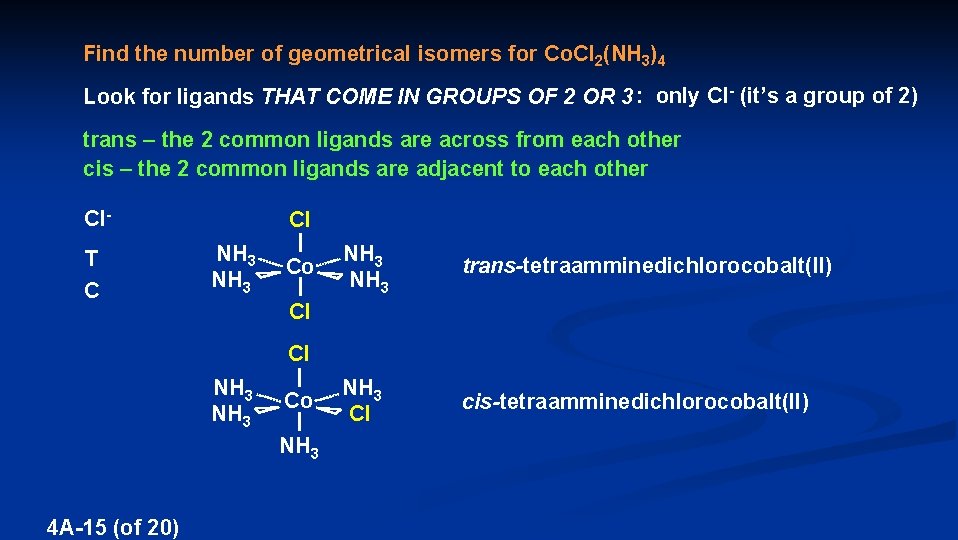
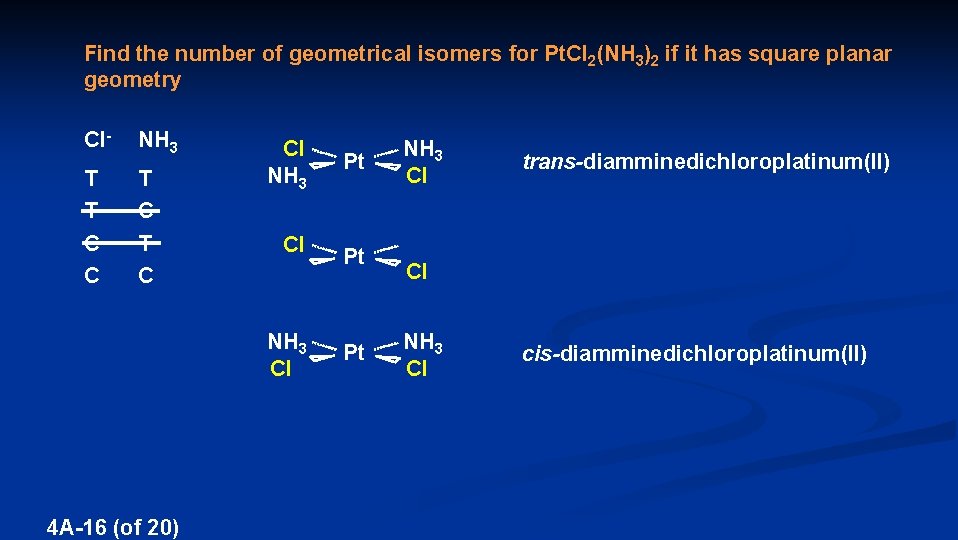
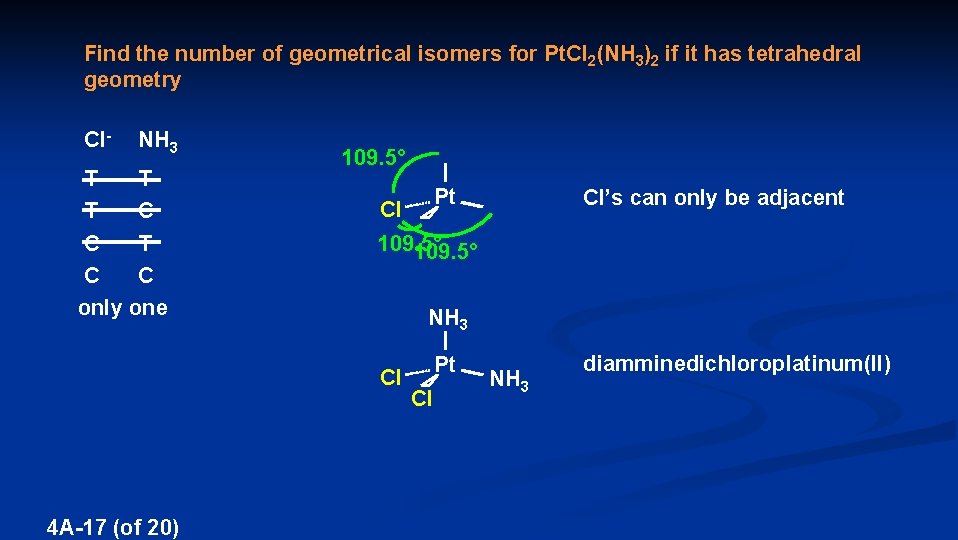
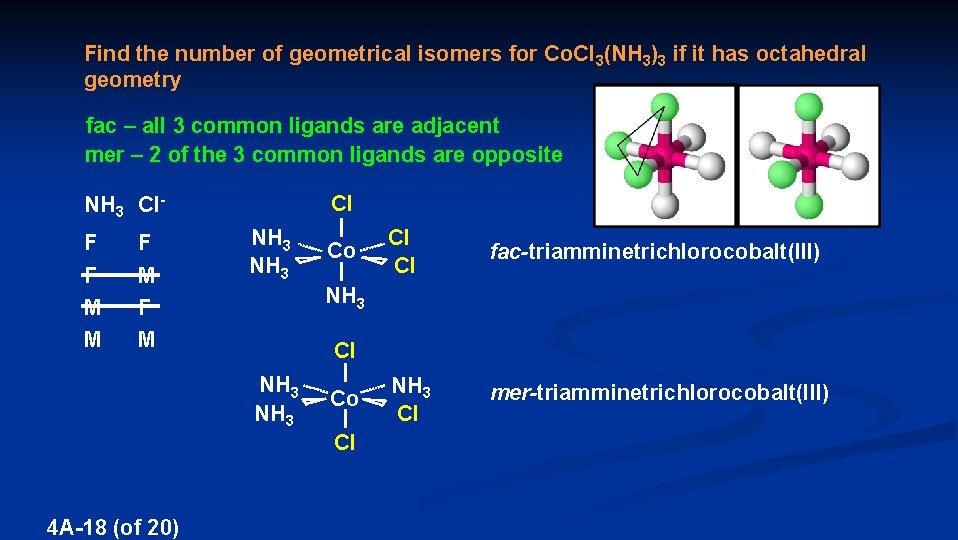
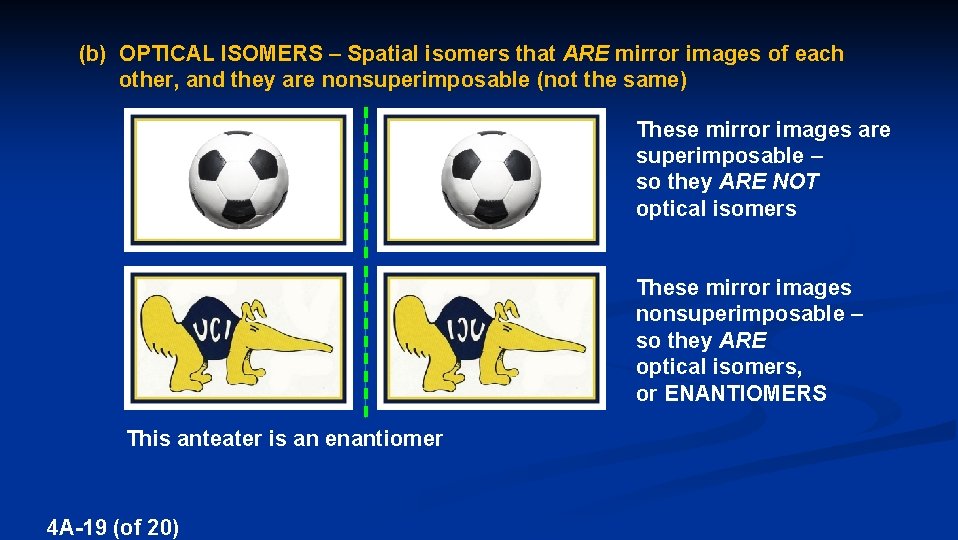
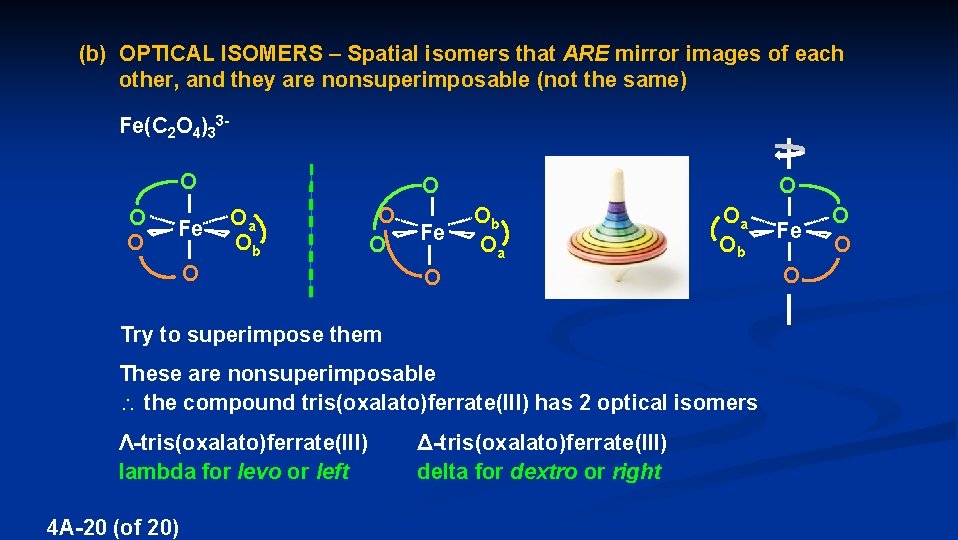
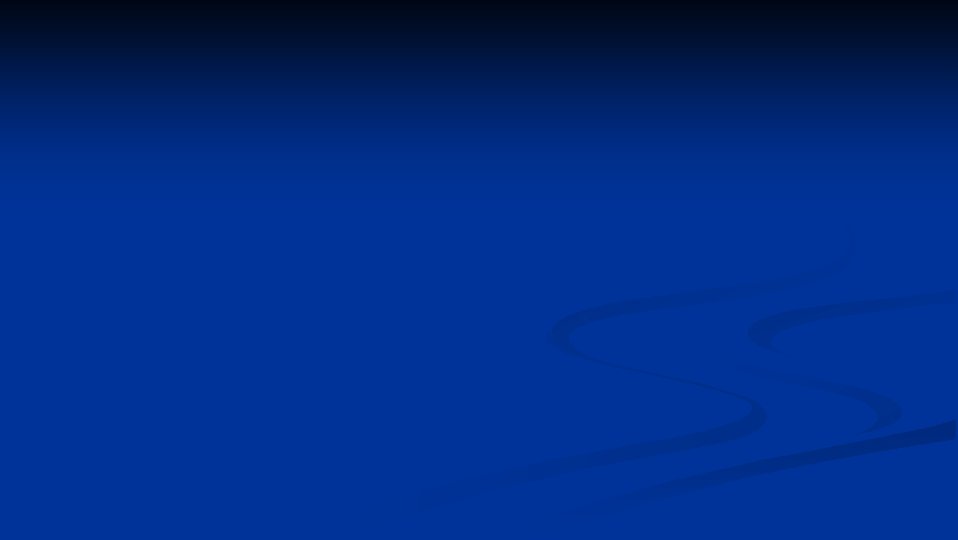
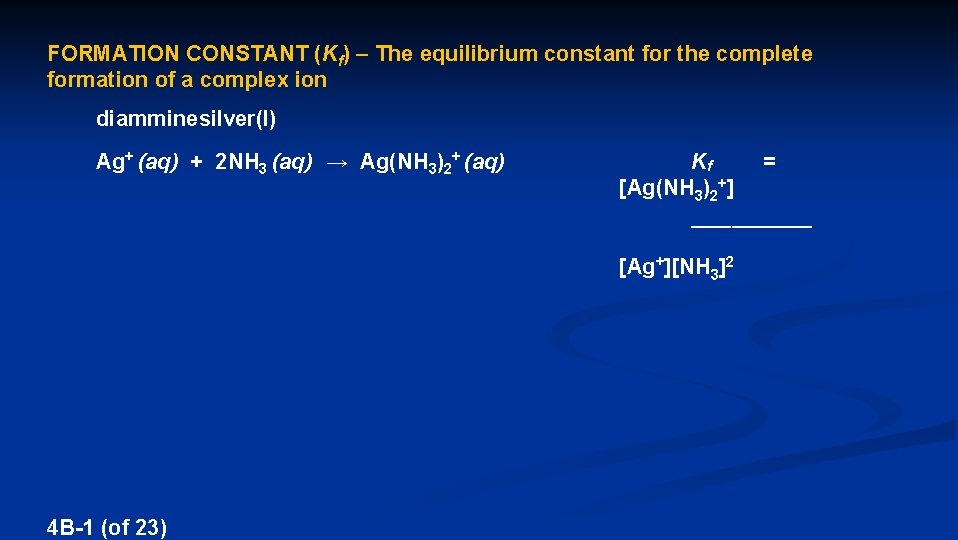
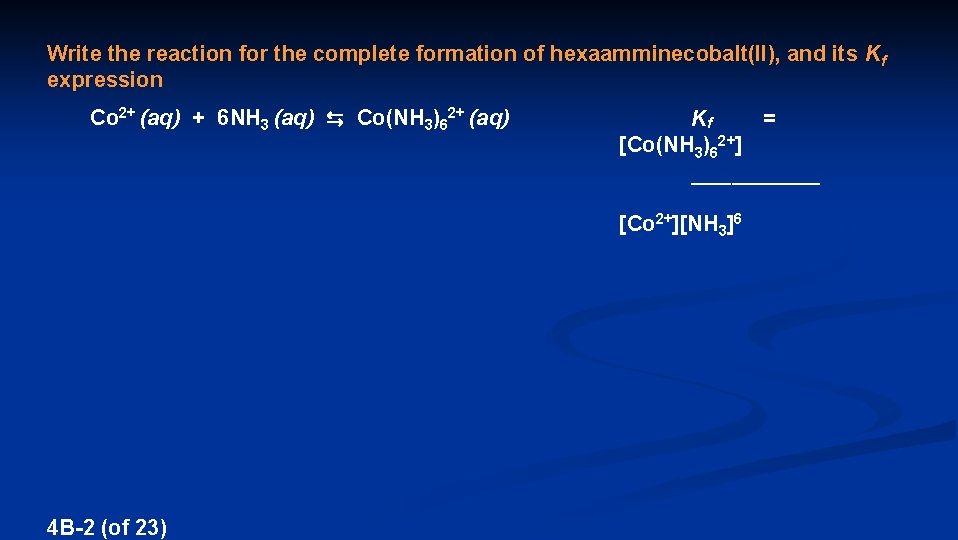
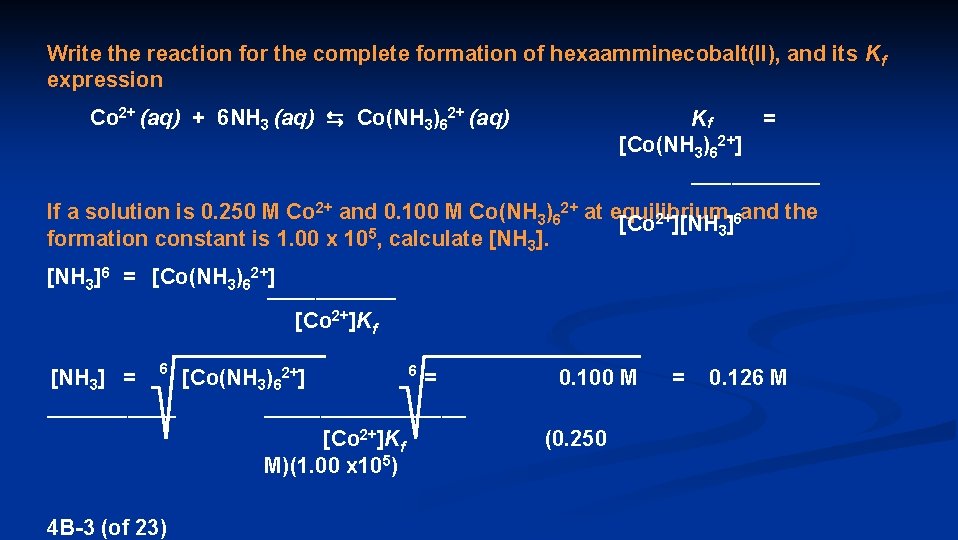
![The formation constant for diamminesilver(I) is 1. 00 x 106. Calculate [Ag+] in a The formation constant for diamminesilver(I) is 1. 00 x 106. Calculate [Ag+] in a](https://slidetodoc.com/presentation_image_h/20ac91025ecf151ea91f5edf1dffa241/image-28.jpg)
![The formation constant for diamminesilver(I) is 1. 00 x 106. Calculate [Ag+] in a The formation constant for diamminesilver(I) is 1. 00 x 106. Calculate [Ag+] in a](https://slidetodoc.com/presentation_image_h/20ac91025ecf151ea91f5edf1dffa241/image-29.jpg)
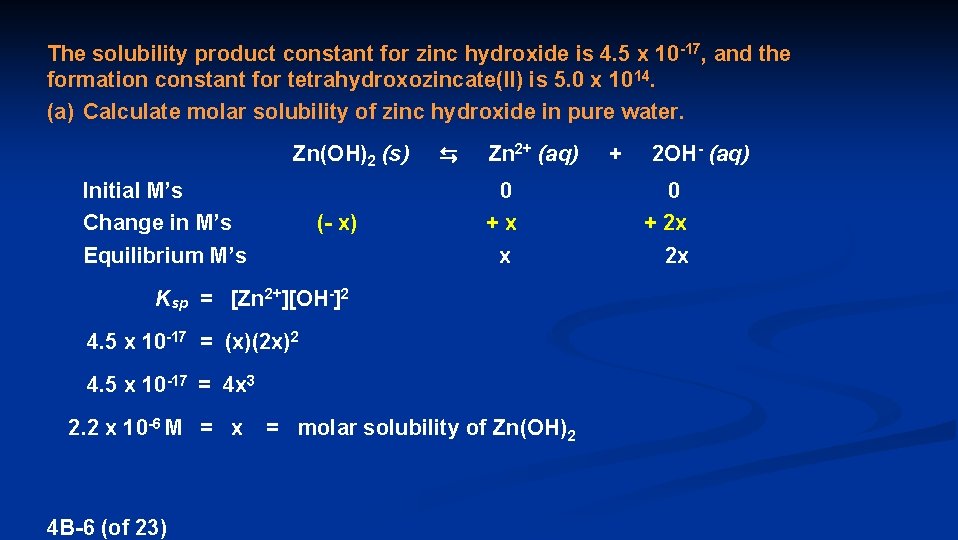
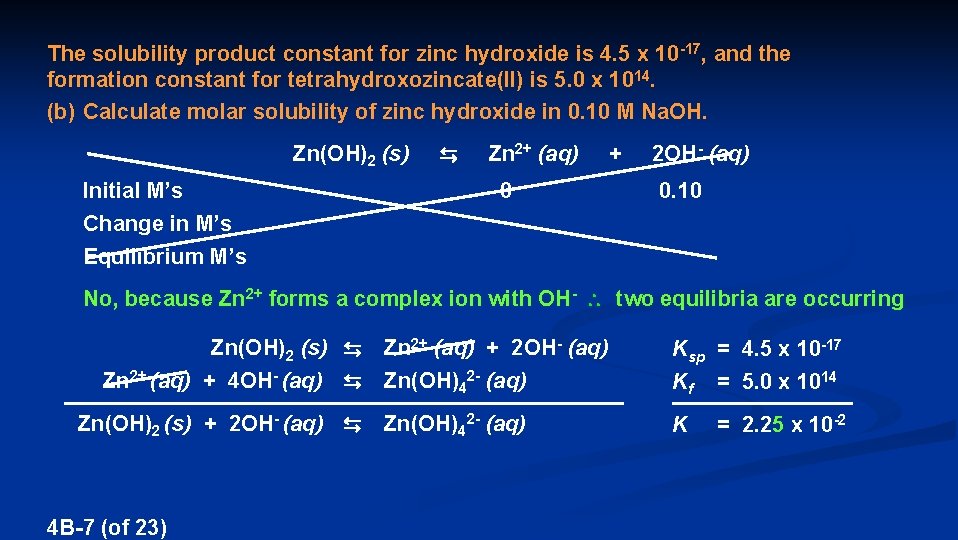
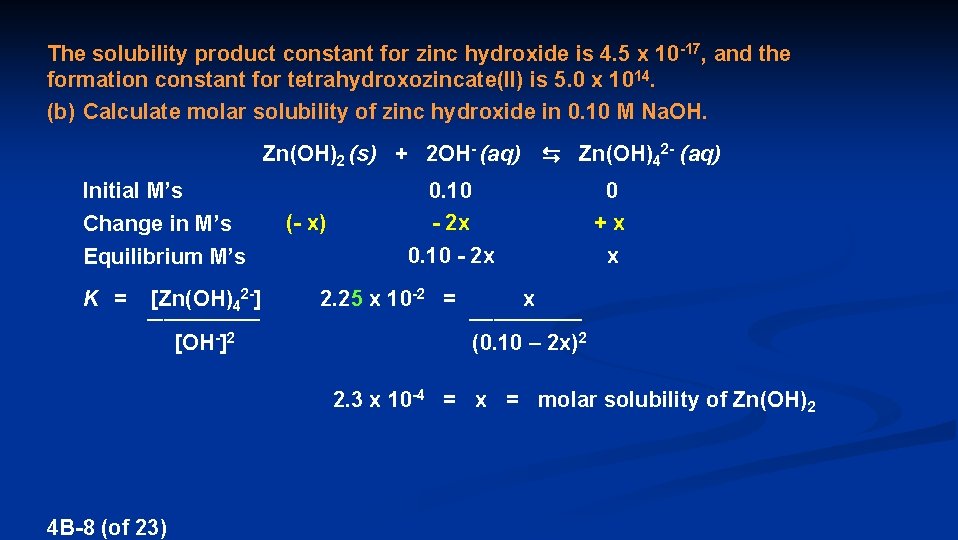
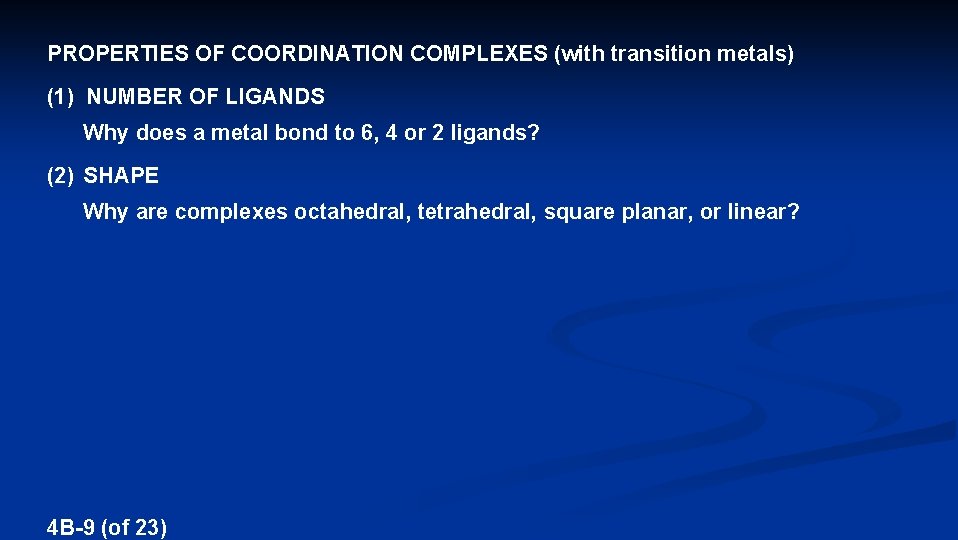
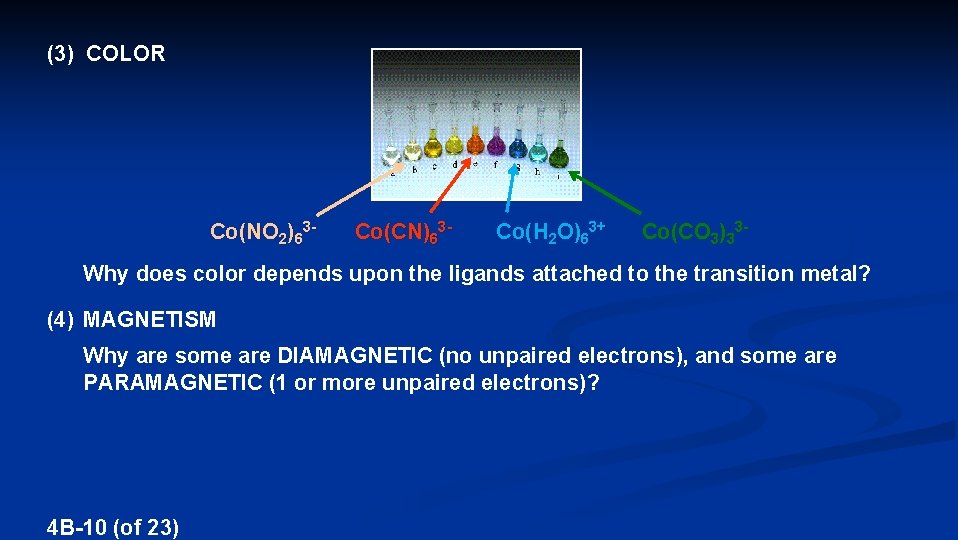
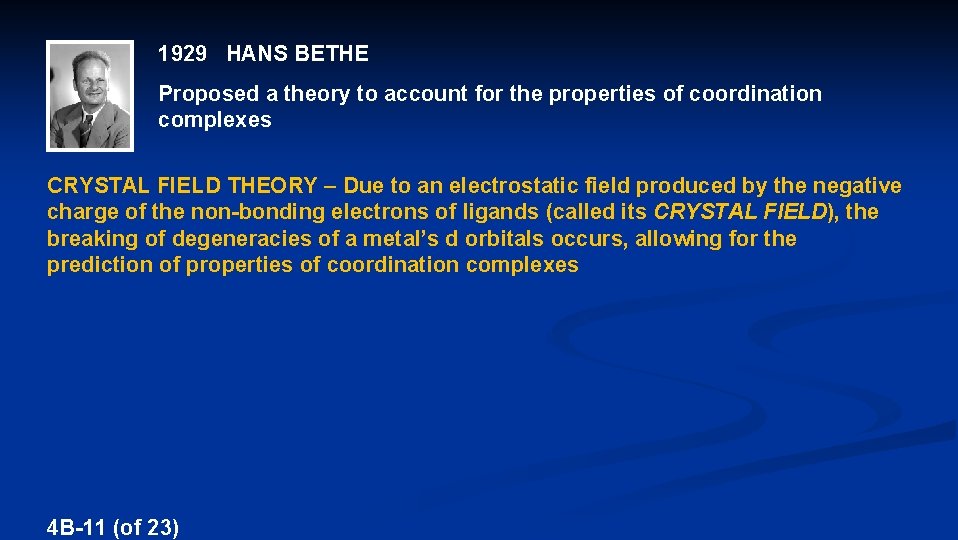
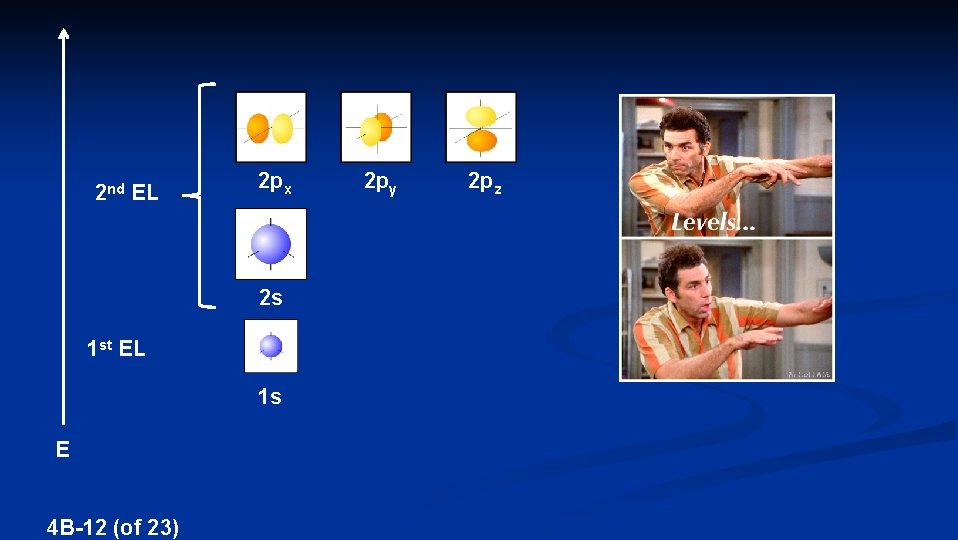
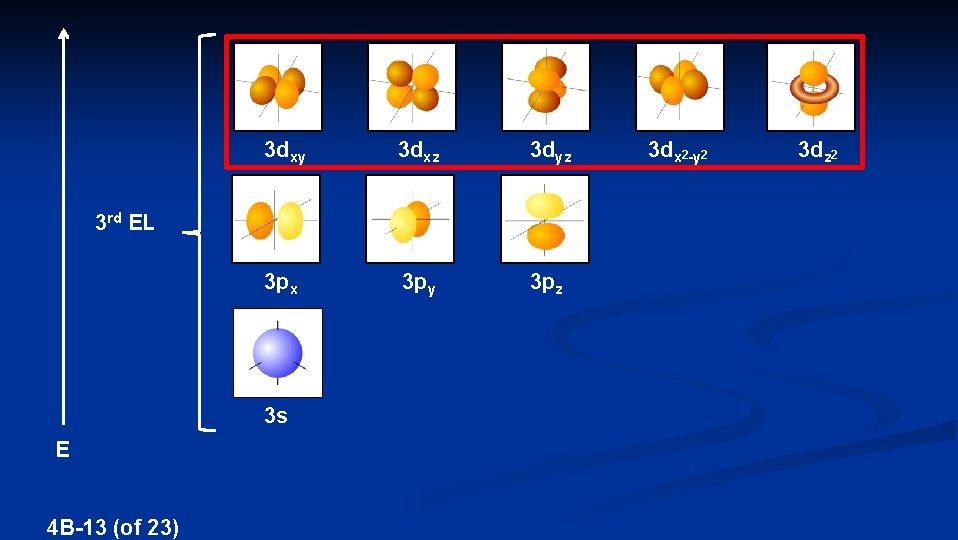
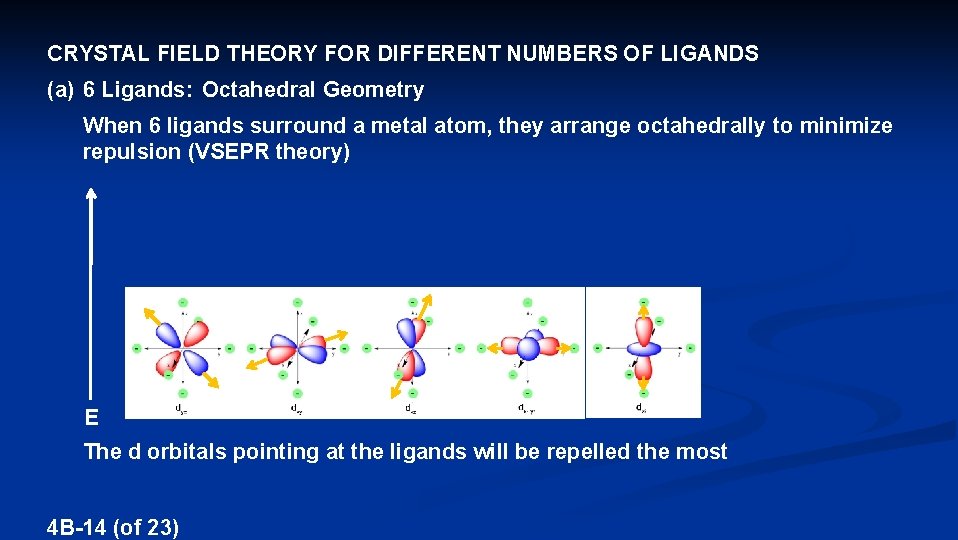
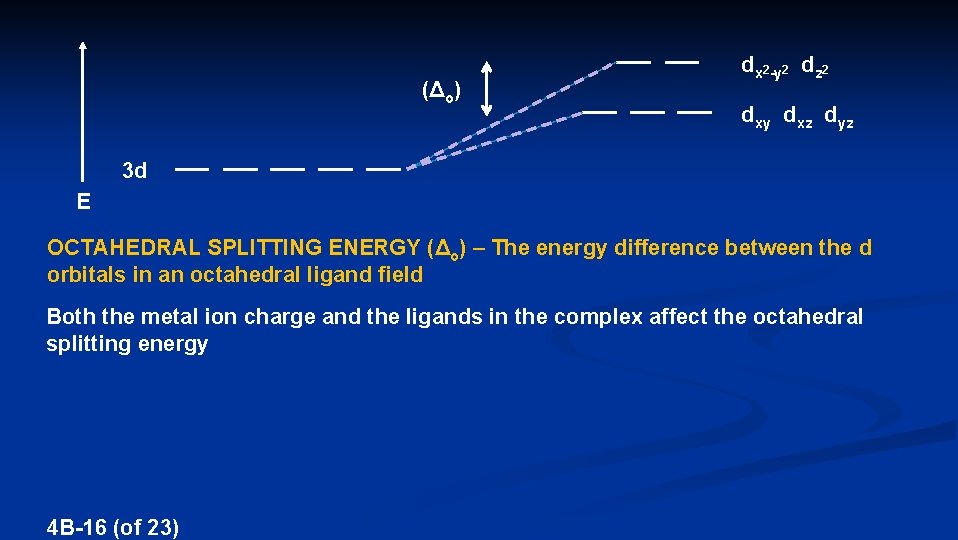
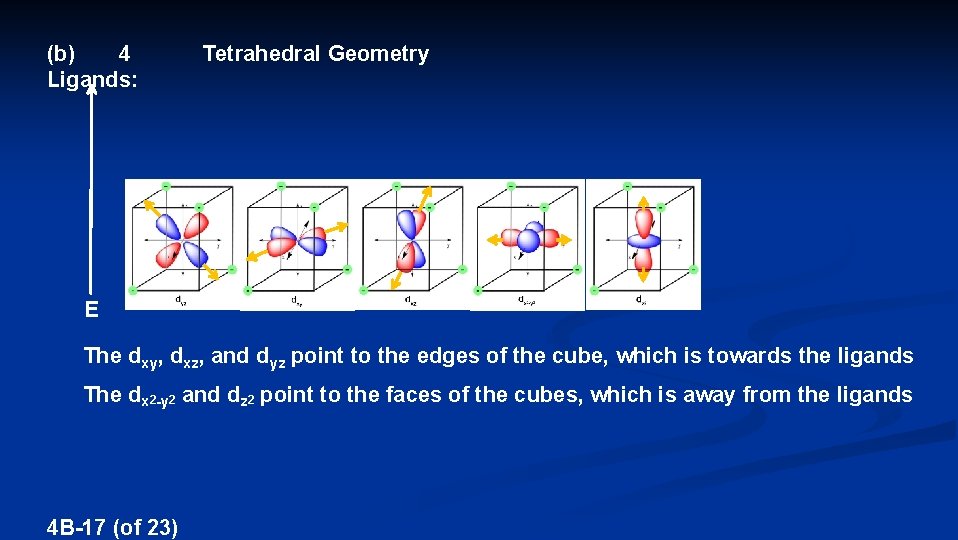
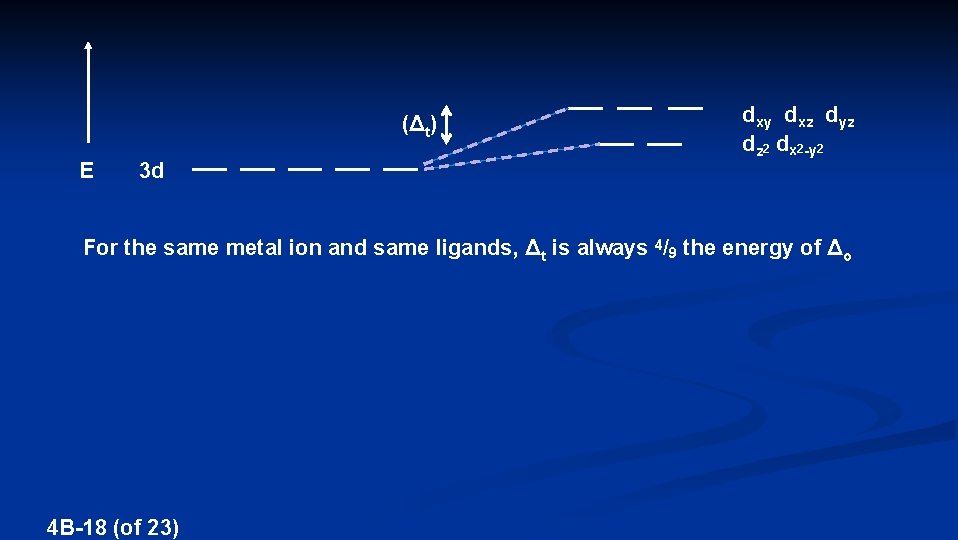
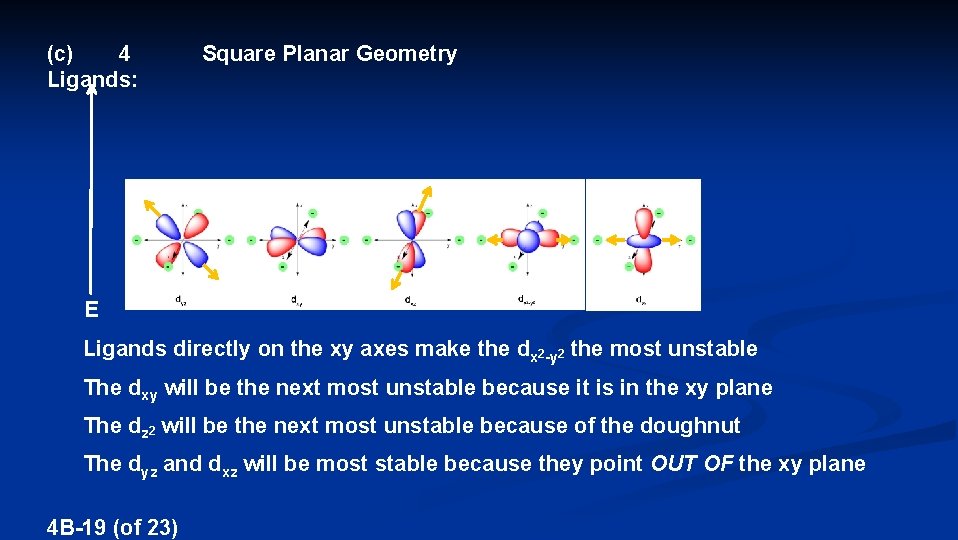
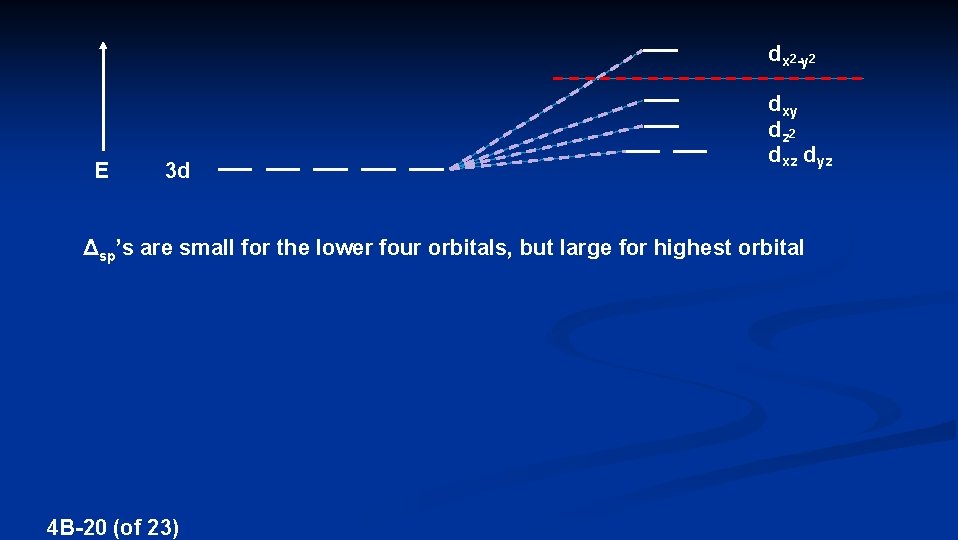
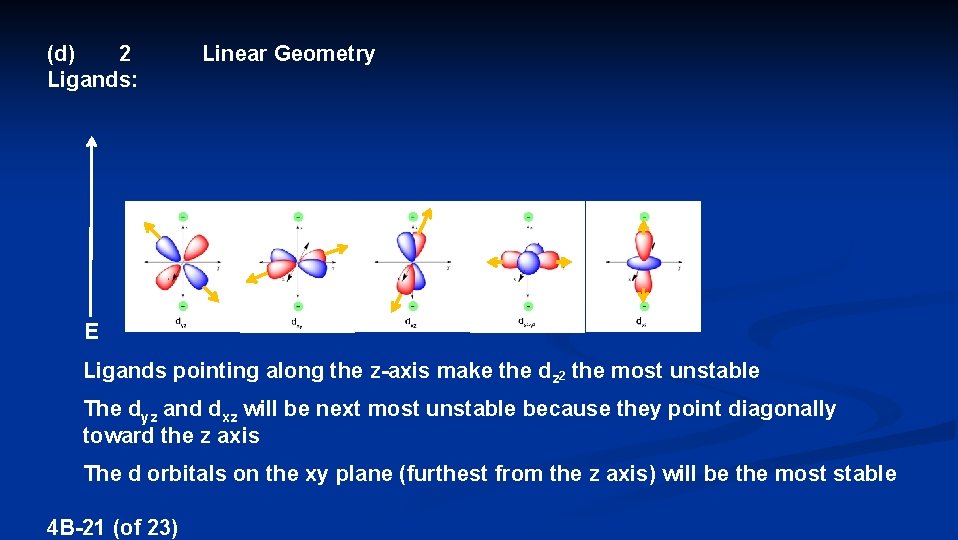
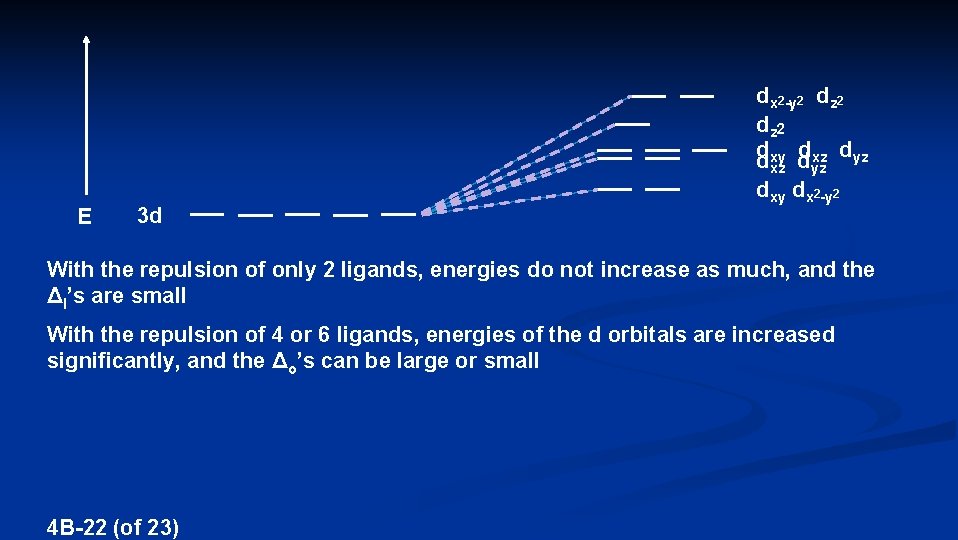
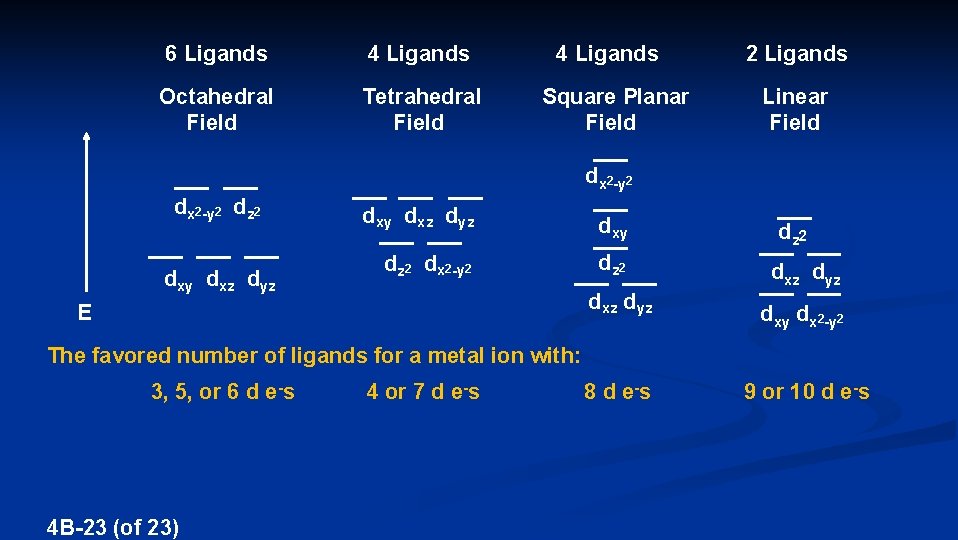
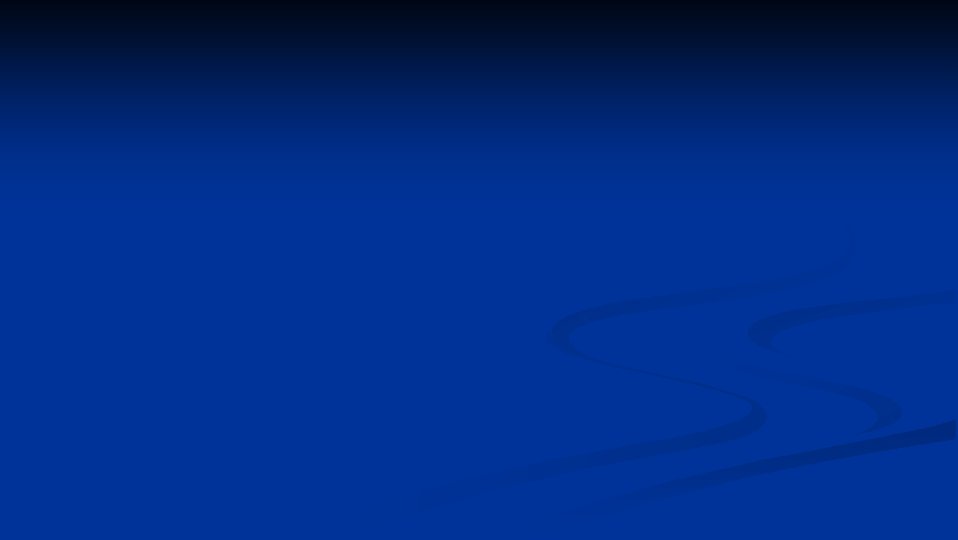
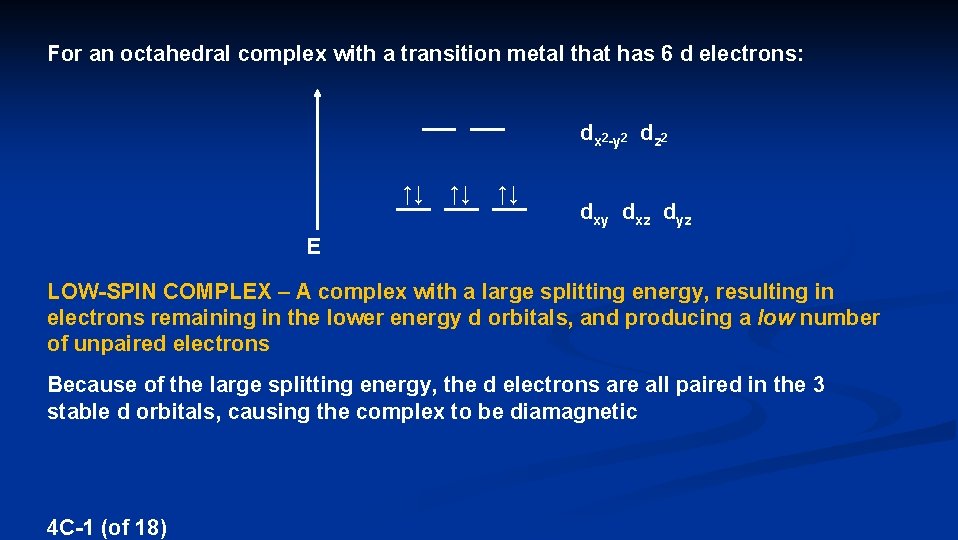
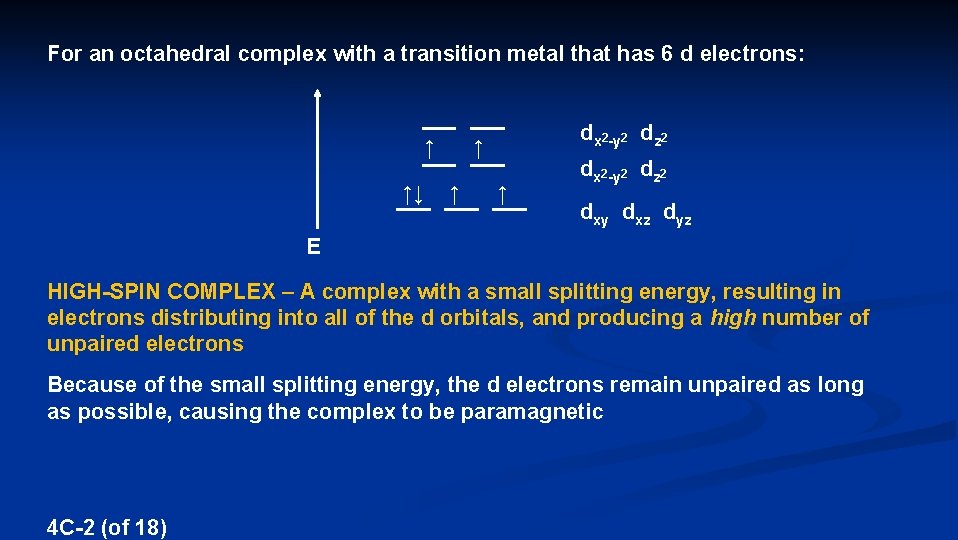
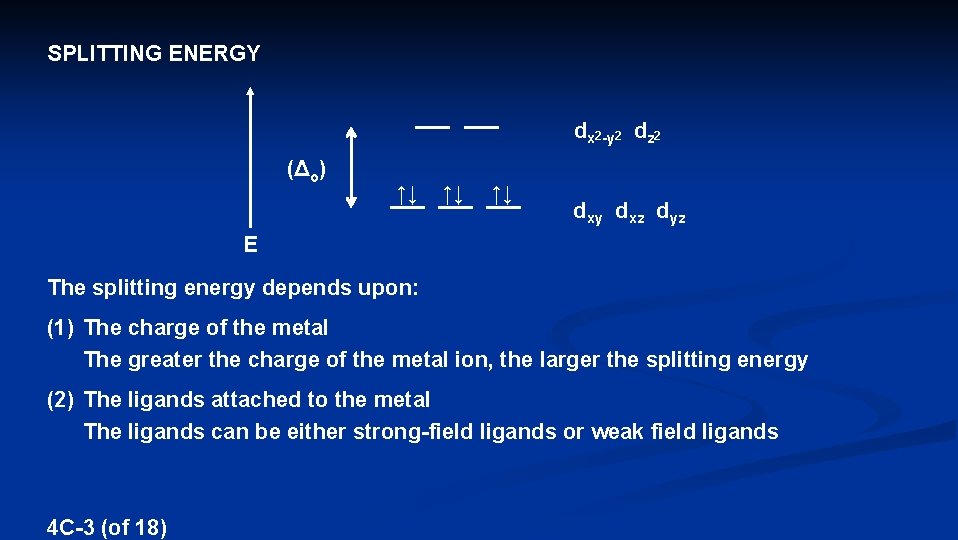
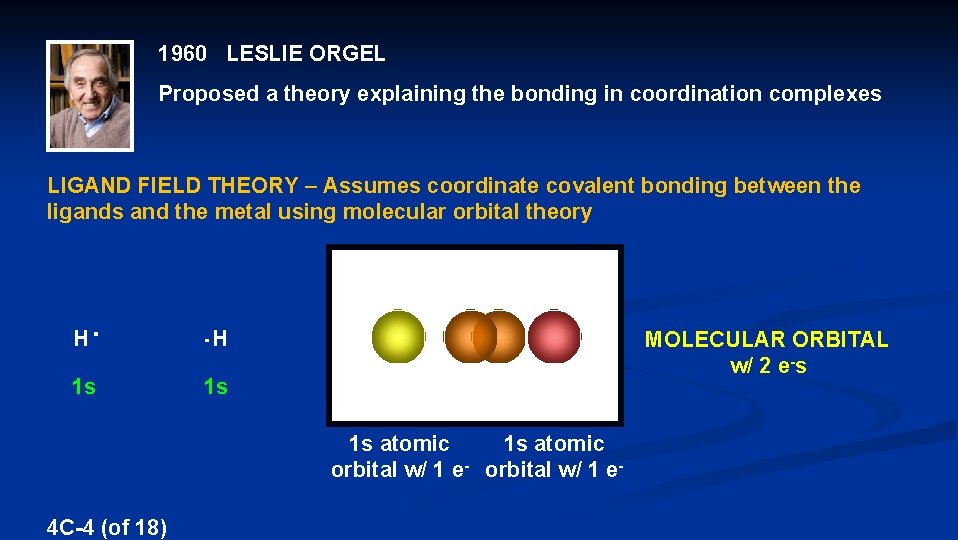
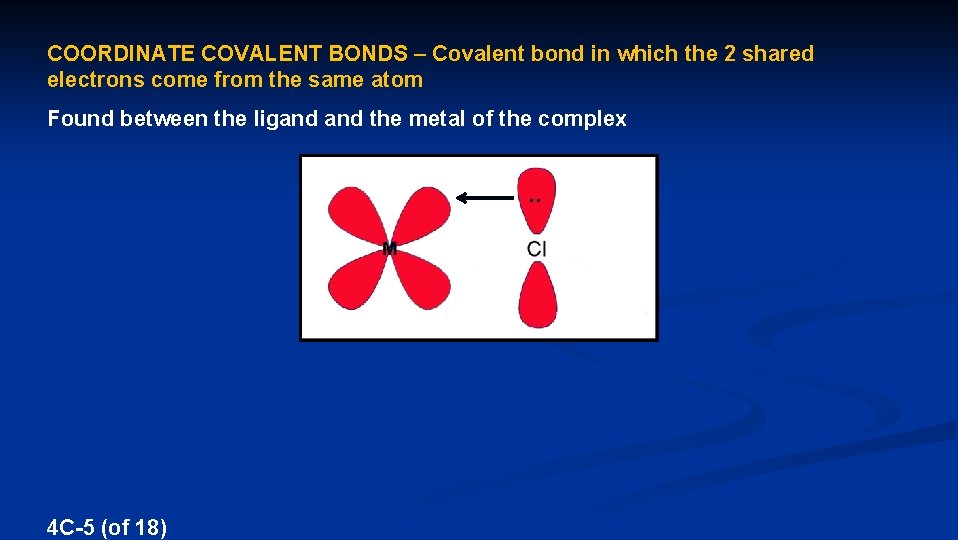
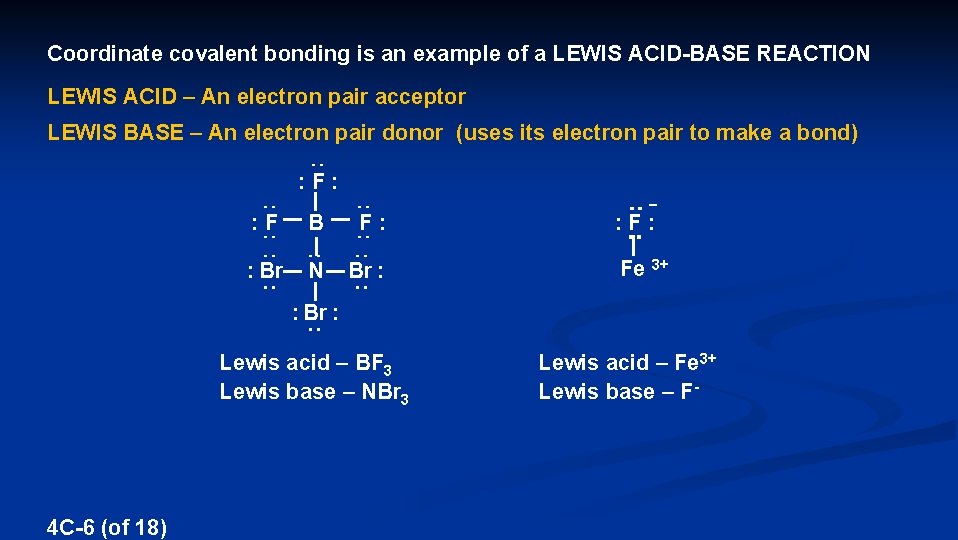
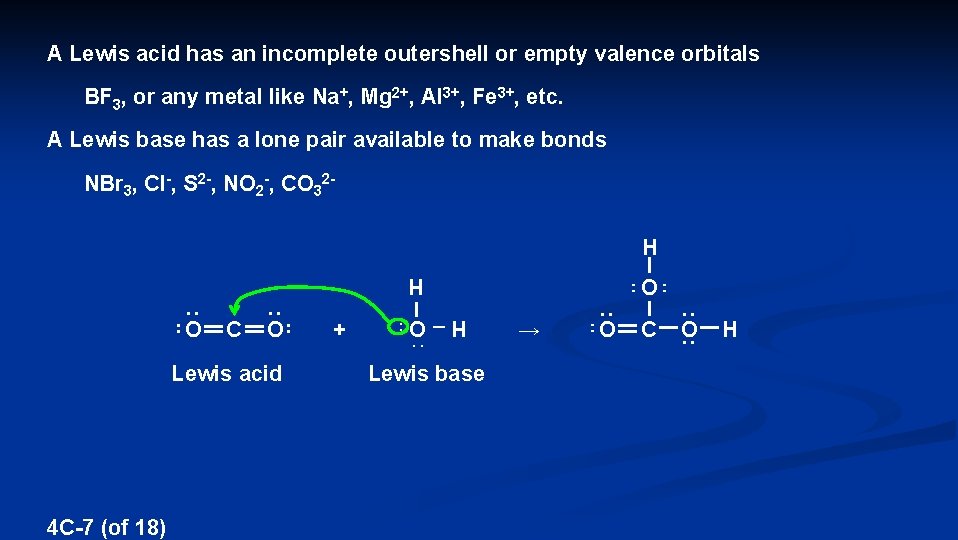
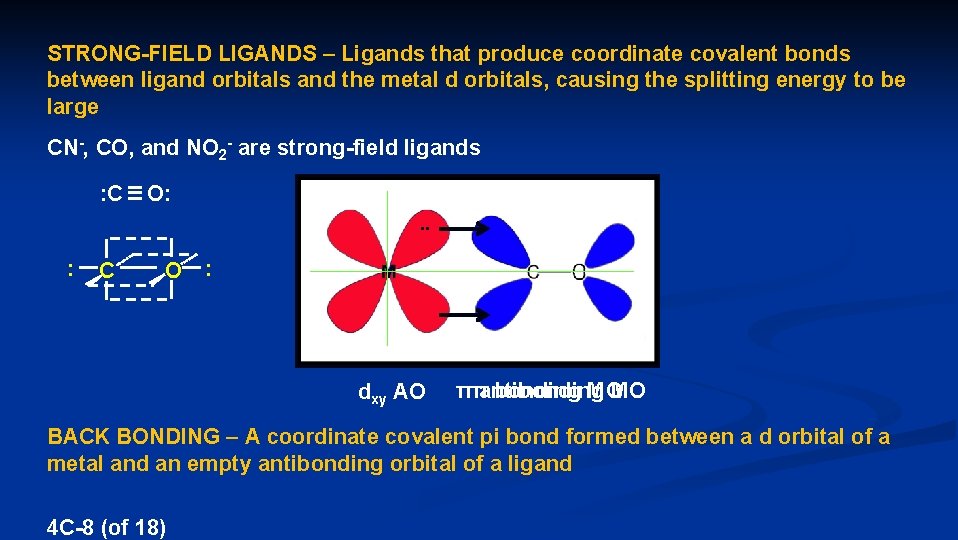
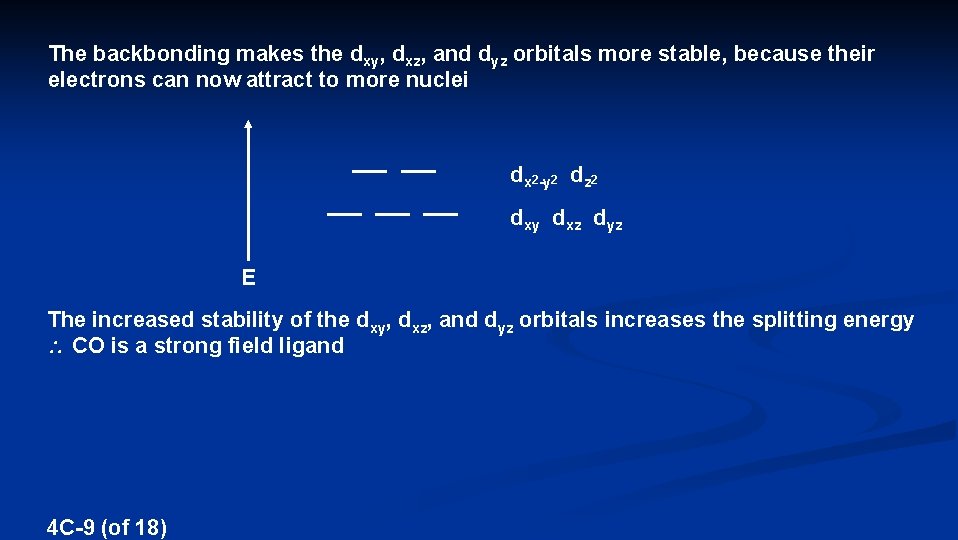
![Fe(CO)64 - 4 C-10 (of 18) hexacarbonylferrate(II) Fe 2+: [Ar]3 d 6 Fe(CO)64 - 4 C-10 (of 18) hexacarbonylferrate(II) Fe 2+: [Ar]3 d 6](https://slidetodoc.com/presentation_image_h/20ac91025ecf151ea91f5edf1dffa241/image-57.jpg)
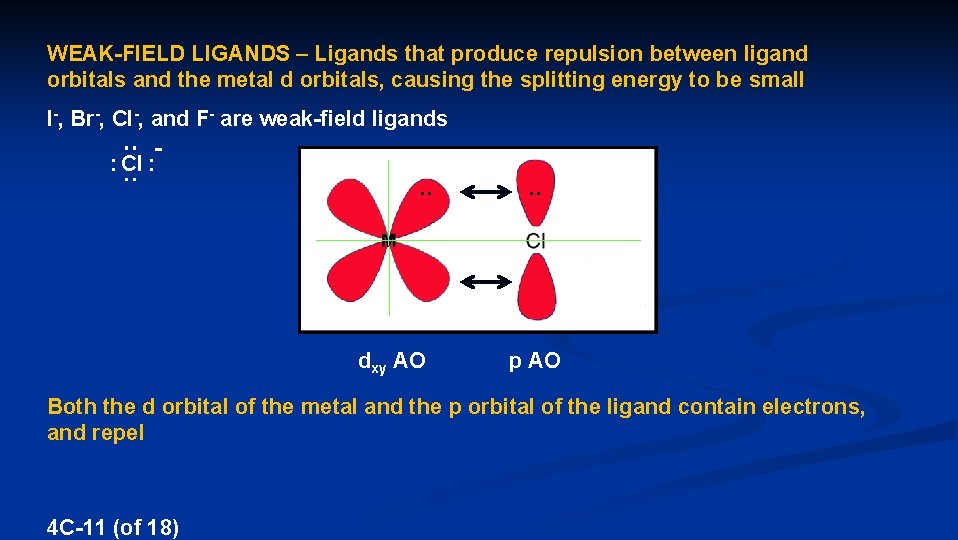
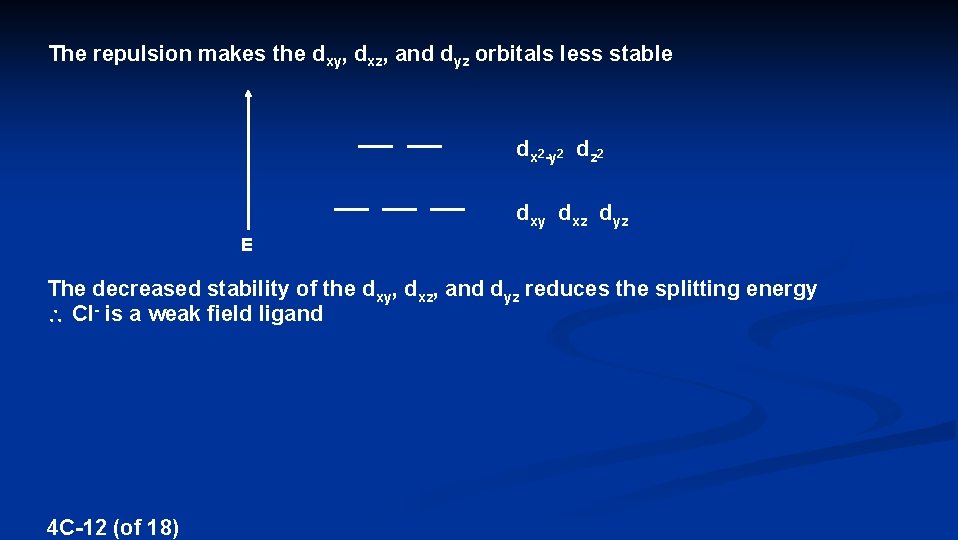
![Fe. Cl 64 - 4 C-13 (of 18) hexachloroferrate(II) Fe 2+: [Ar]3 d 6 Fe. Cl 64 - 4 C-13 (of 18) hexachloroferrate(II) Fe 2+: [Ar]3 d 6](https://slidetodoc.com/presentation_image_h/20ac91025ecf151ea91f5edf1dffa241/image-60.jpg)
![Hexafluorocobaltate(III) (a) Give the electron configuration of the cobalt ion Co atom: [Ar]4 s Hexafluorocobaltate(III) (a) Give the electron configuration of the cobalt ion Co atom: [Ar]4 s](https://slidetodoc.com/presentation_image_h/20ac91025ecf151ea91f5edf1dffa241/image-61.jpg)
![Hexacarbonyliron(II) (a) Give the electron configuration of the iron ion Fe atom: [Ar]4 s Hexacarbonyliron(II) (a) Give the electron configuration of the iron ion Fe atom: [Ar]4 s](https://slidetodoc.com/presentation_image_h/20ac91025ecf151ea91f5edf1dffa241/image-62.jpg)
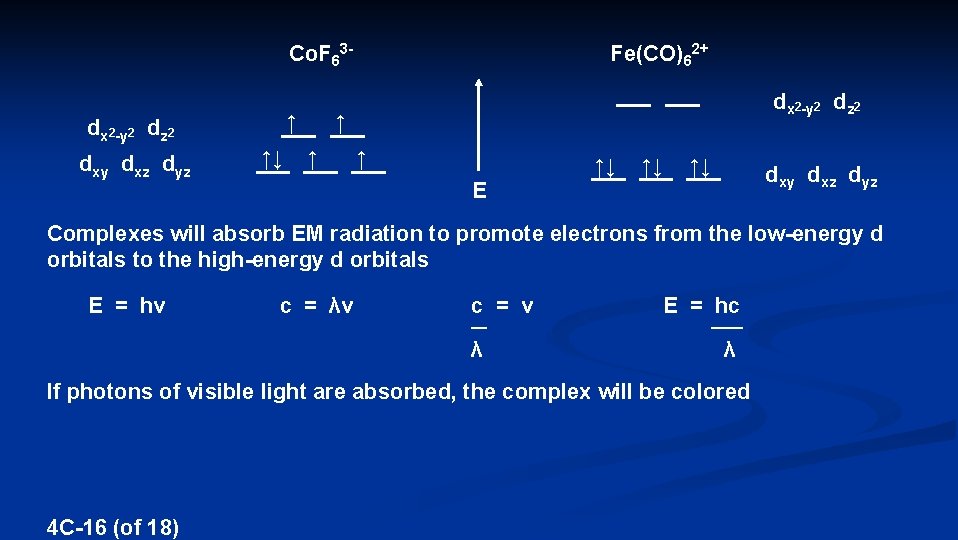
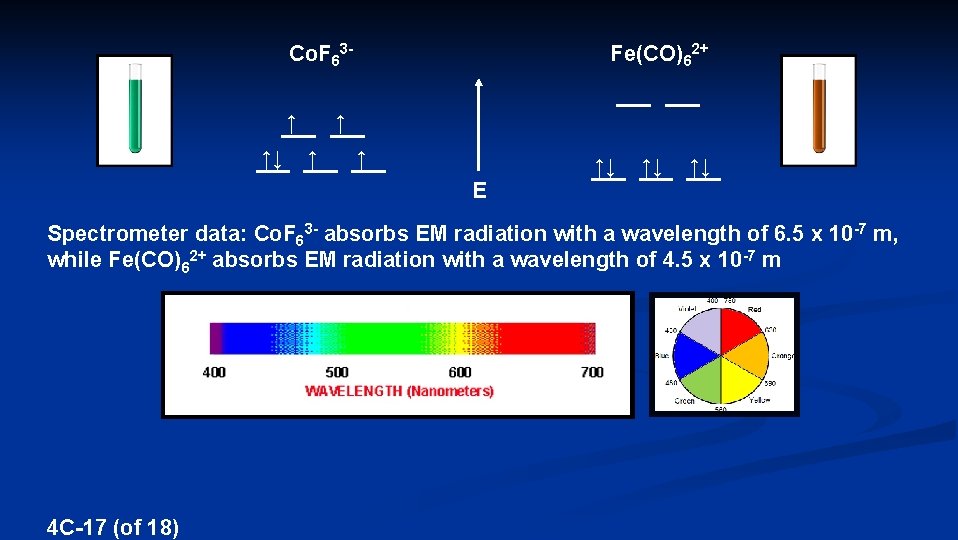
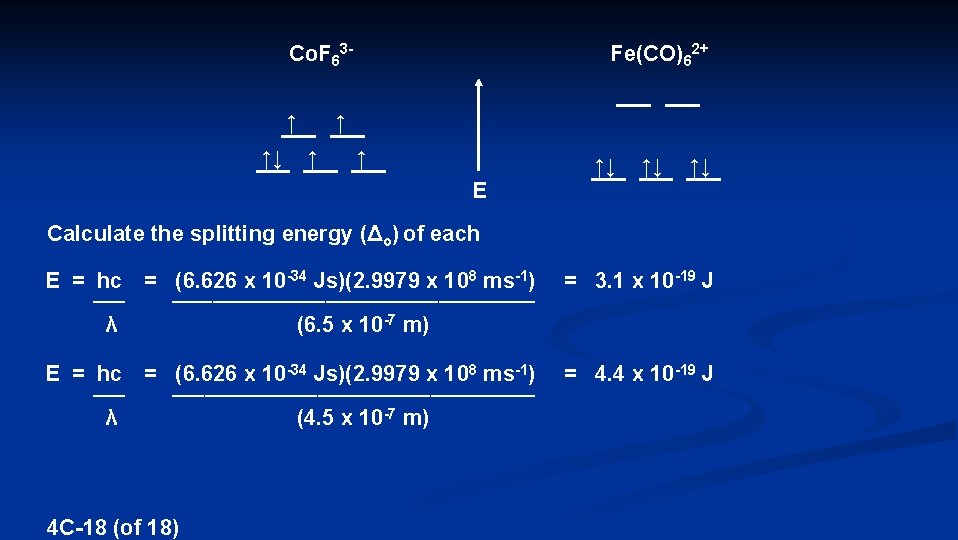
- Slides: 65
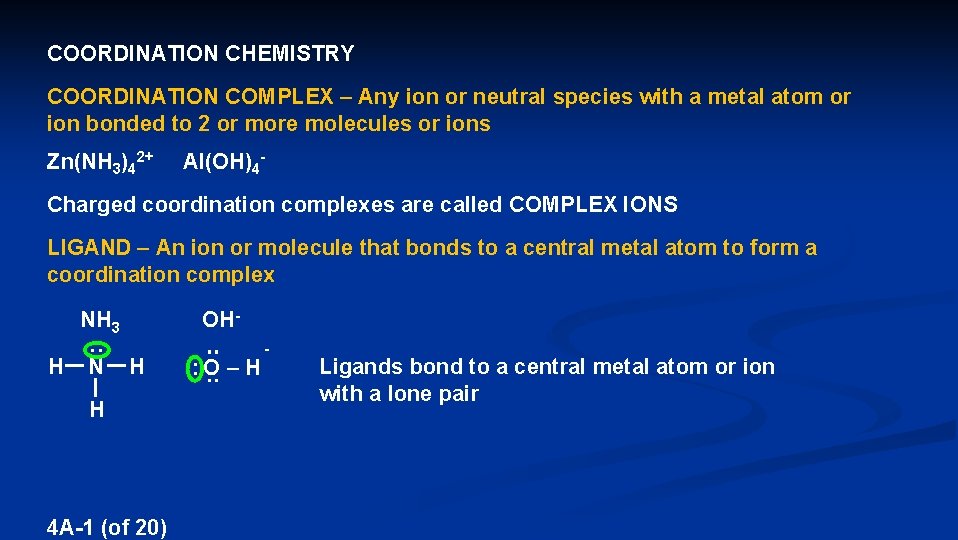
COORDINATION CHEMISTRY COORDINATION COMPLEX – Any ion or neutral species with a metal atom or ion bonded to 2 or more molecules or ions Zn(NH 3)42+ Al(OH)4 - Charged coordination complexes are called COMPLEX IONS LIGAND – An ion or molecule that bonds to a central metal atom to form a coordination complex NH 3 H N H OH : O – H H 4 A-1 (of 20) Ligands bond to a central metal atom or ion with a lone pair
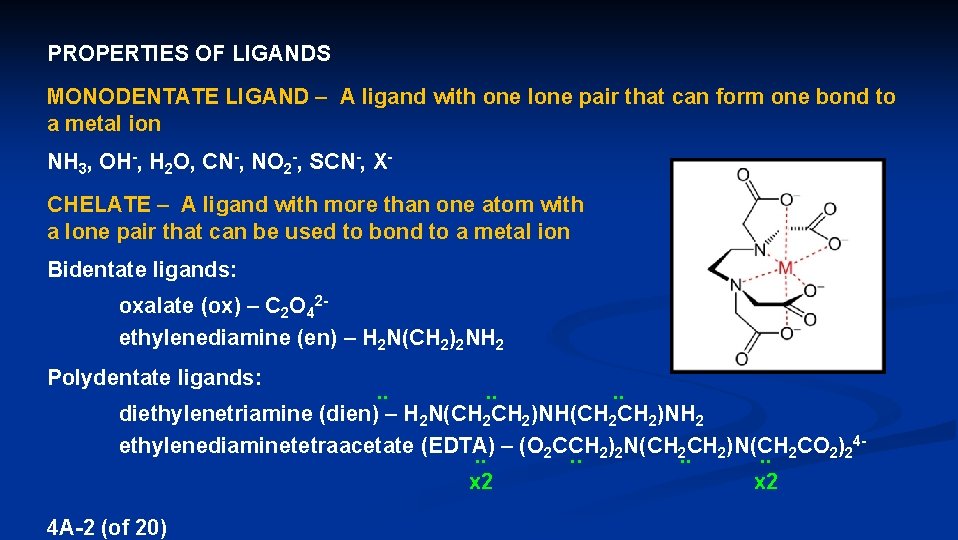
PROPERTIES OF LIGANDS MONODENTATE LIGAND – A ligand with one lone pair that can form one bond to a metal ion NH 3, OH-, H 2 O, CN-, NO 2 -, SCN-, XCHELATE – A ligand with more than one atom with a lone pair that can be used to bond to a metal ion Bidentate ligands: oxalate (ox) – C 2 O 42 - ethylenediamine (en) – H 2 N(CH 2)2 NH 2 Polydentate ligands: . . . . diethylenetriamine (dien) – H 2 N(CH 2 CH 2)NH 2 4 ethylenediaminetetraacetate (EDTA) – (O CCH ) N(CH CH )N(CH CO ) 2 2 2 2 . . x 2 x 2 4 A-2 (of 20)
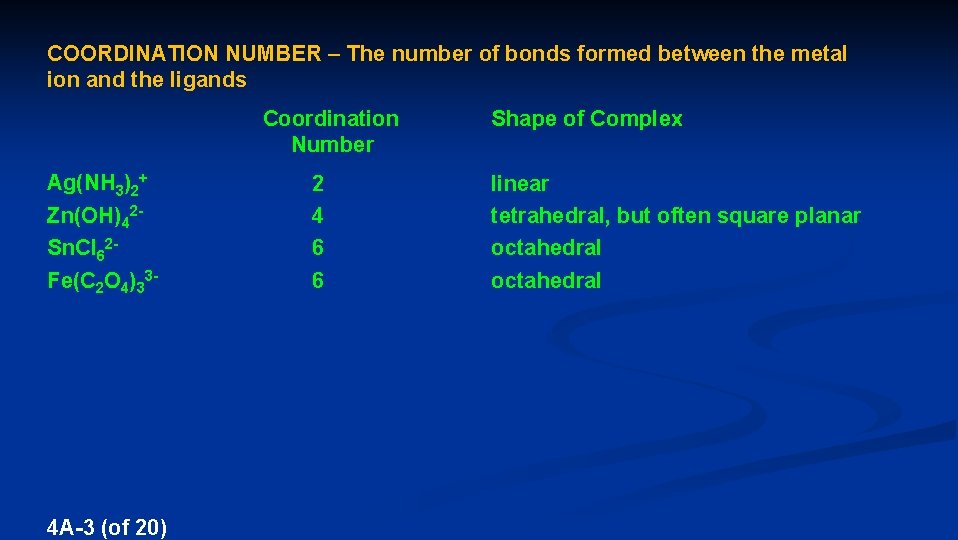
COORDINATION NUMBER – The number of bonds formed between the metal ion and the ligands Coordination Number Ag(NH 3)2+ Zn(OH)42 Sn. Cl 62 - Fe(C 2 O 4)33 - 4 A-3 (of 20) 2 4 6 6 Shape of Complex linear tetrahedral, but often square planar octahedral
![COORDINATION COMPOUND Any compound containing a complex ion and a counterion CuNH 34Cl COORDINATION COMPOUND – Any compound containing a complex ion and a counterion [Cu(NH 3)4]Cl](https://slidetodoc.com/presentation_image_h/20ac91025ecf151ea91f5edf1dffa241/image-4.jpg)
COORDINATION COMPOUND – Any compound containing a complex ion and a counterion [Cu(NH 3)4]Cl 2 This is a 2+ charged complex ion, requiring 2 Cl- counterions to produce a neutral compound 4 A-4 (of 20)
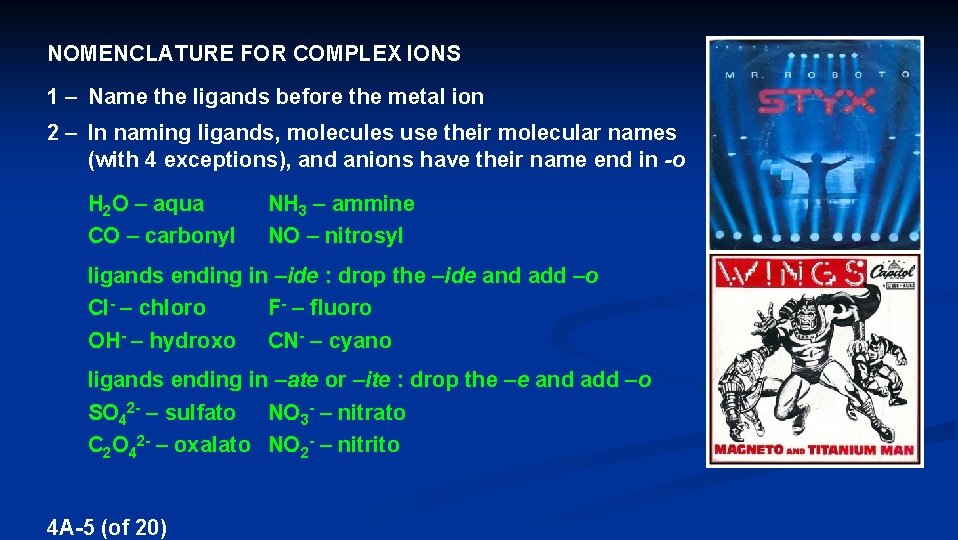
NOMENCLATURE FOR COMPLEX IONS 1 – Name the ligands before the metal ion 2 – In naming ligands, molecules use their molecular names (with 4 exceptions), and anions have their name end in -o H 2 O – aqua CO – carbonyl NH 3 – ammine NO – nitrosyl ligands ending in –ide : drop the –ide and add –o Cl- – chloro F- – fluoro OH- – hydroxo CN- – cyano ligands ending in –ate or –ite : drop the –e and add –o SO 42 - – sulfato NO 3 - – nitrato C 2 O 42 - – oxalato NO 2 - – nitrito 4 A-5 (of 20)
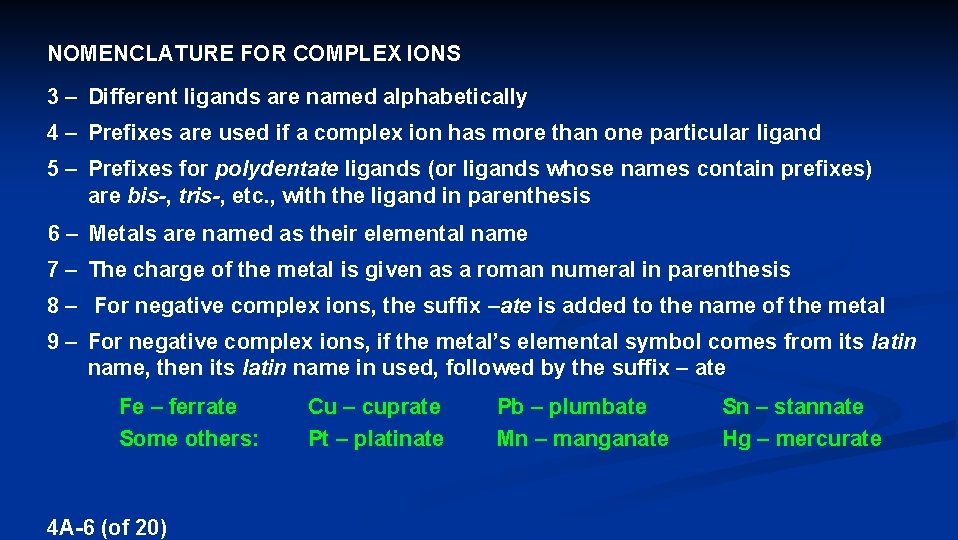
NOMENCLATURE FOR COMPLEX IONS 3 – Different ligands are named alphabetically 4 – Prefixes are used if a complex ion has more than one particular ligand 5 – Prefixes for polydentate ligands (or ligands whose names contain prefixes) are bis-, tris-, etc. , with the ligand in parenthesis 6 – Metals are named as their elemental name 7 – The charge of the metal is given as a roman numeral in parenthesis 8 – For negative complex ions, the suffix –ate is added to the name of the metal 9 – For negative complex ions, if the metal’s elemental symbol comes from its latin name, then its latin name in used, followed by the suffix – ate Fe – ferrate Some others: 4 A-6 (of 20) Cu – cuprate Pt – platinate Pb – plumbate Mn – manganate Sn – stannate Hg – mercurate
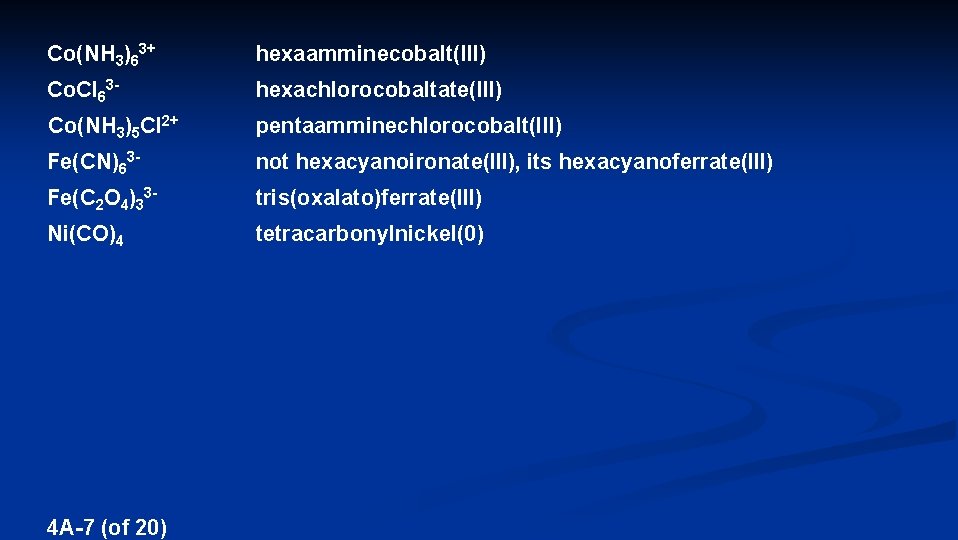
Co(NH 3)63+ hexaamminecobalt(III) Co. Cl 63 - hexachlorocobaltate(III) Co(NH 3)5 Cl 2+ pentaamminechlorocobalt(III) Fe(CN)63 - not hexacyanoironate(III), its hexacyanoferrate(III) Fe(C 2 O 4)33 - tris(oxalato)ferrate(III) Ni(CO)4 tetracarbonylnickel(0) 4 A-7 (of 20)
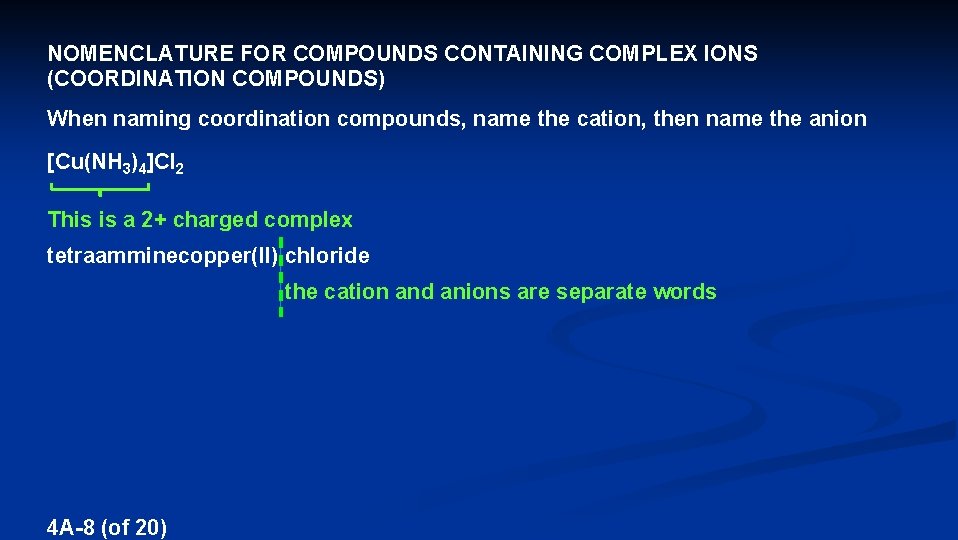
NOMENCLATURE FOR COMPOUNDS CONTAINING COMPLEX IONS (COORDINATION COMPOUNDS) When naming coordination compounds, name the cation, then name the anion [Cu(NH 3)4]Cl 2 This is a 2+ charged complex tetraamminecopper(II) chloride the cation and anions are separate words 4 A-8 (of 20)
![CrNH 36Cl 3 This is a 3 charged complex ion hexaamminechromiumIII chloride PtNH 33 [Cr(NH 3)6]Cl 3 This is a 3+ charged complex ion hexaamminechromium(III) chloride [Pt(NH 3)3](https://slidetodoc.com/presentation_image_h/20ac91025ecf151ea91f5edf1dffa241/image-9.jpg)
[Cr(NH 3)6]Cl 3 This is a 3+ charged complex ion hexaamminechromium(III) chloride [Pt(NH 3)3 Cl 3]Cl This is a 1+ charged complex ion triamminetrichloroplatinum(IV) chloride Mn(en)2 Cl 2 This is a neutral complex dichlorobis(ethylenediamine)manganese(II) 4 A-9 (of 20)
![KPt NH 3 Cl 5 This is a 1 charged complex ion potassium K[Pt. NH 3 Cl 5] This is a 1 - charged complex ion potassium](https://slidetodoc.com/presentation_image_h/20ac91025ecf151ea91f5edf1dffa241/image-10.jpg)
K[Pt. NH 3 Cl 5] This is a 1 - charged complex ion potassium amminepentachloroplatinate(IV) K 4 Fe(CN)6 This is a 4 - charged complex ion potassium hexacyanoferrate(II) [Fe(en)2(NO 2)2]2 SO 4 This is a 1+ complex ion bis(ethylenediamine)dinitritoiron(III) sulfate 4 A-10 (of 20)
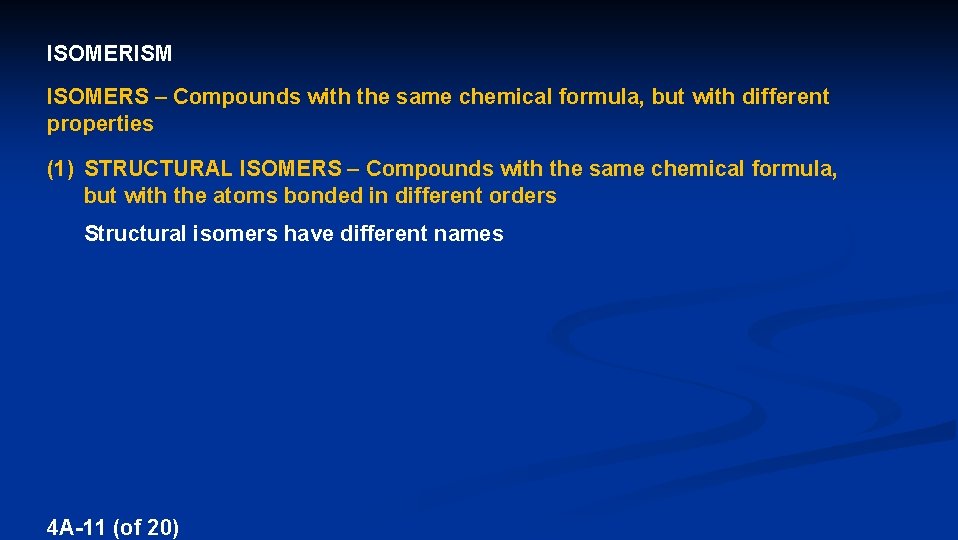
ISOMERISM ISOMERS – Compounds with the same chemical formula, but with different properties (1) STRUCTURAL ISOMERS – Compounds with the same chemical formula, but with the atoms bonded in different orders Structural isomers have different names 4 A-11 (of 20)
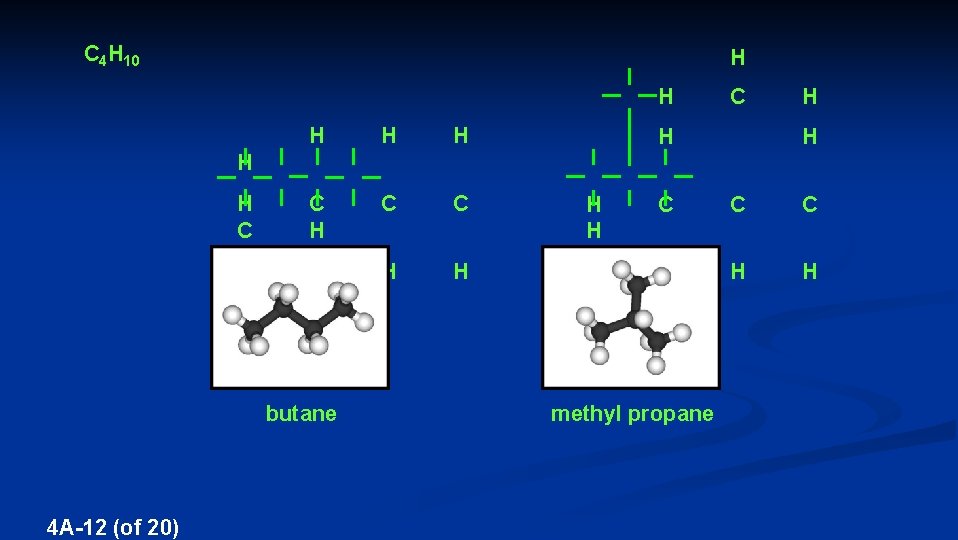
C 4 H 10 H H H C C H H H H H C H H C C C H H butane 4 A-12 (of 20) methyl propane
![PtH 2 O4OH2 Cl 2 PtH 2 O4OH2Cl 2 H 2 O Pt 2 Pt(H 2 O)4(OH)2 Cl 2 [Pt(H 2 O)4(OH)2]Cl 2 H 2 O Pt 2+](https://slidetodoc.com/presentation_image_h/20ac91025ecf151ea91f5edf1dffa241/image-13.jpg)
Pt(H 2 O)4(OH)2 Cl 2 [Pt(H 2 O)4(OH)2]Cl 2 H 2 O Pt 2+ OH OH Cl- H 2 O [Pt(H 2 O)4 Cl 2](OH)2 H 2 O tetraaquadihydroxoplatinum(IV) chloride Cl Pt Cl H 2 O 4 A-13 (of 20) tetraaquadichloroplatinum(IV) hydroxide 2+ OH-
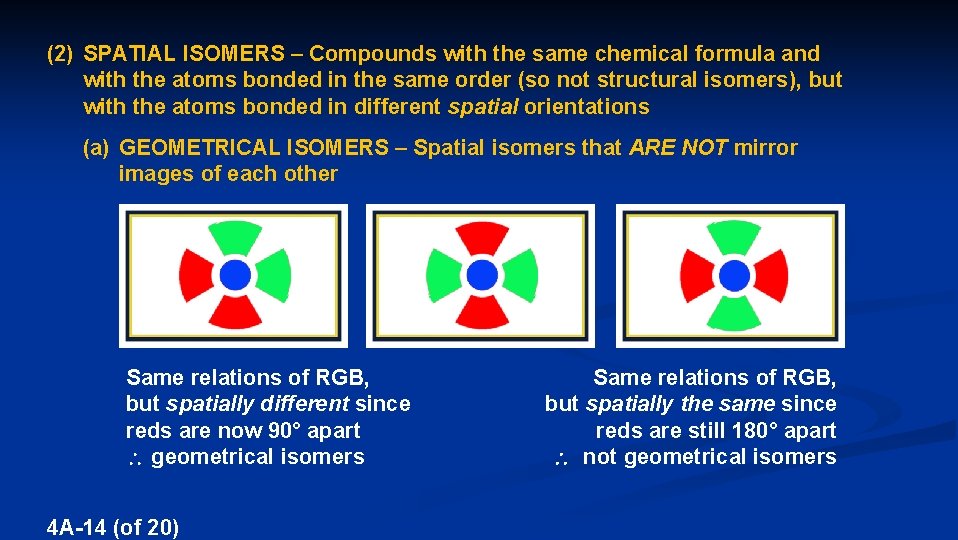
(2) SPATIAL ISOMERS – Compounds with the same chemical formula and with the atoms bonded in the same order (so not structural isomers), but with the atoms bonded in different spatial orientations (a) GEOMETRICAL ISOMERS – Spatial isomers that ARE NOT mirror images of each other Same relations of RGB, but spatially different since reds are now 90° apart geometrical isomers 4 A-14 (of 20) Same relations of RGB, but spatially the same since reds are still 180° apart not geometrical isomers
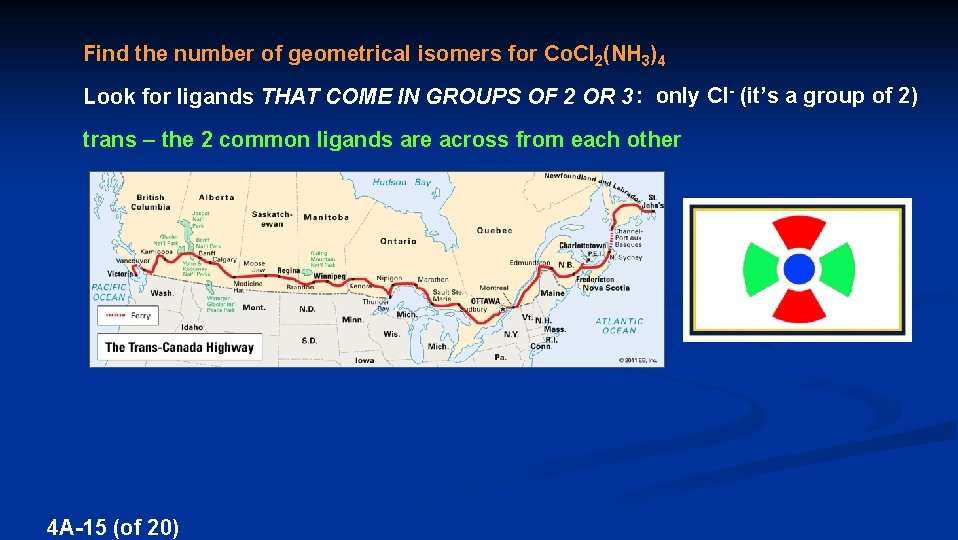
Find the number of geometrical isomers for Co. Cl 2(NH 3)4 Look for ligands THAT COME IN GROUPS OF 2 OR 3 : only Cl- (it’s a group of 2) trans – the 2 common ligands are across from each other 4 A-15 (of 20)
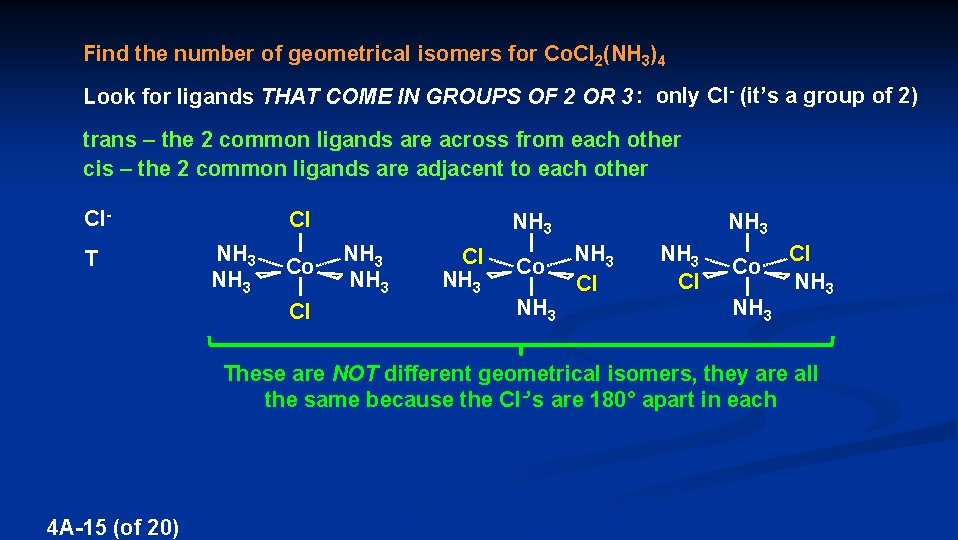
Find the number of geometrical isomers for Co. Cl 2(NH 3)4 Look for ligands THAT COME IN GROUPS OF 2 OR 3 : only Cl- (it’s a group of 2) trans – the 2 common ligands are across from each other cis – the 2 common ligands are adjacent to each other Cl- Cl T NH 3 Cl Co NH 3 Cl NH 3 Co NH 3 NH 3 Cl Co NH 3 Cl NH 3 These are NOT different geometrical isomers, they are all the same because the Cl-’s are 180° apart in each 4 A-15 (of 20)
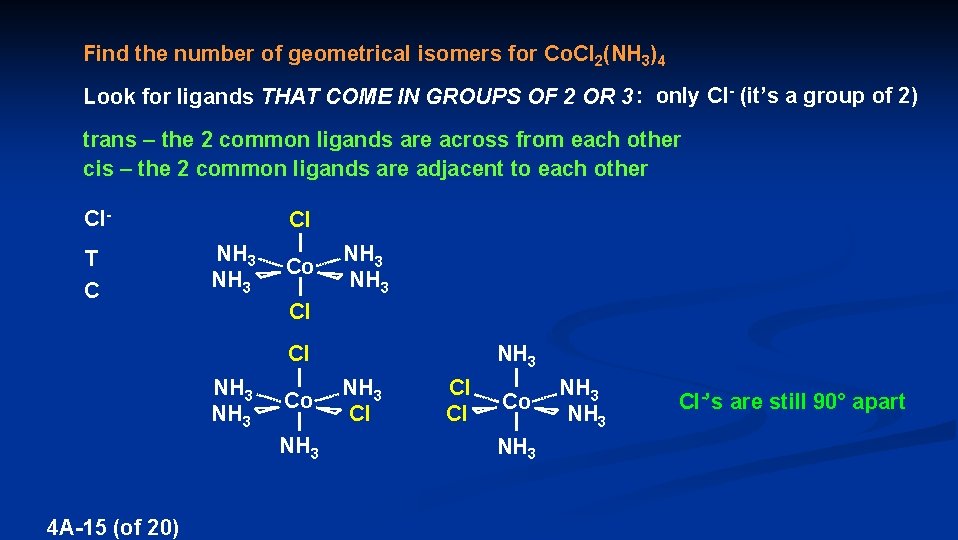
Find the number of geometrical isomers for Co. Cl 2(NH 3)4 Look for ligands THAT COME IN GROUPS OF 2 OR 3 : only Cl- (it’s a group of 2) trans – the 2 common ligands are across from each other cis – the 2 common ligands are adjacent to each other Cl. T C Cl NH 3 Cl Co Cl NH 3 4 A-15 (of 20) NH 3 Co Cl NH 3 Cl Cl NH 3 Co NH 3 Cl-’s are still 90° apart
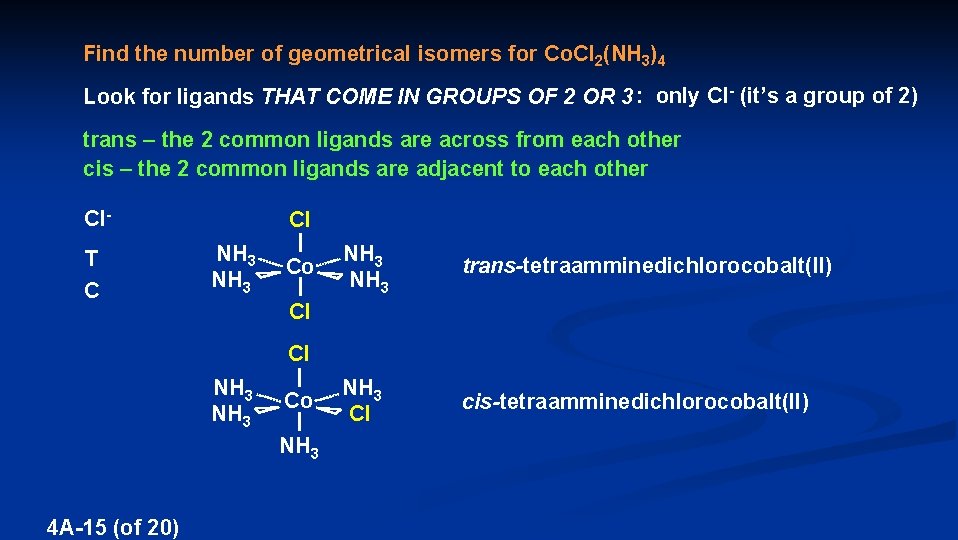
Find the number of geometrical isomers for Co. Cl 2(NH 3)4 Look for ligands THAT COME IN GROUPS OF 2 OR 3 : only Cl- (it’s a group of 2) trans – the 2 common ligands are across from each other cis – the 2 common ligands are adjacent to each other Cl. T C Cl NH 3 Cl Co trans-tetraamminedichlorocobalt(II) Cl NH 3 4 A-15 (of 20) NH 3 Co Cl NH 3 cis-tetraamminedichlorocobalt(II)
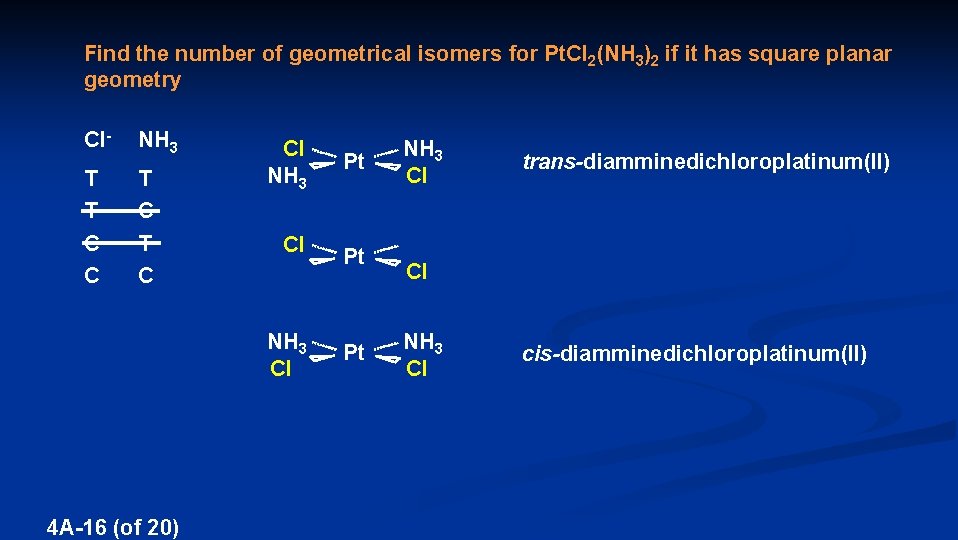
Find the number of geometrical isomers for Pt. Cl 2(NH 3)2 if it has square planar geometry Cl- NH 3 T T C Cl NH 3 Pt NH 3 Cl trans-diamminedichloroplatinum(II) Cl Pt Cl NH 3 Cl Pt 4 A-16 (of 20) NH 3 Cl cis-diamminedichloroplatinum(II)
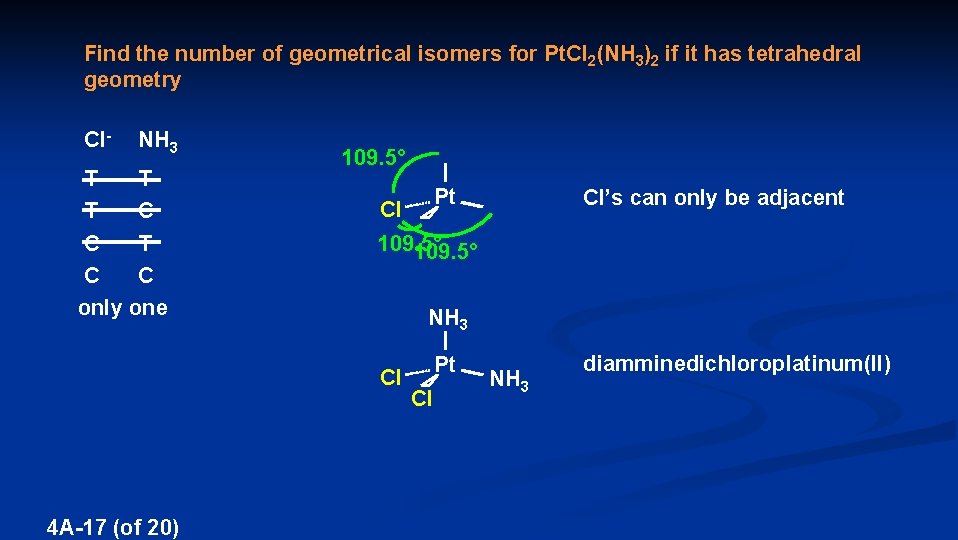
Find the number of geometrical isomers for Pt. Cl 2(NH 3)2 if it has tetrahedral geometry Cl- NH 3 T T T C C only one 109. 5° Pt 109. 5° Cl NH 3 Pt Cl NH 3 Cl 4 A-17 (of 20) Cl’s can only be adjacent diamminedichloroplatinum(II)
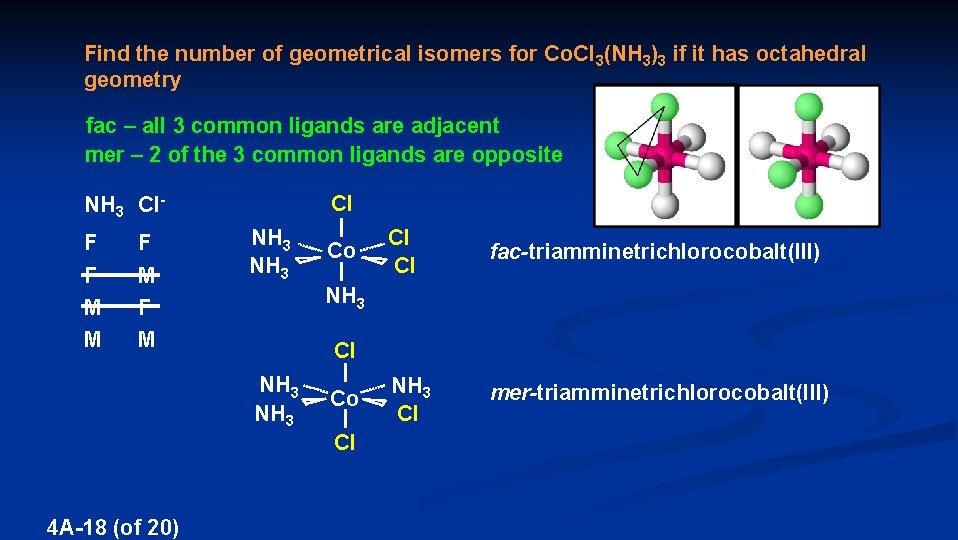
Find the number of geometrical isomers for Co. Cl 3(NH 3)3 if it has octahedral geometry fac – all 3 common ligands are adjacent mer – 2 of the 3 common ligands are opposite Cl NH 3 Cl. F F M M F M NH 3 fac-triamminetrichlorocobalt(III) Cl NH 3 4 A-18 (of 20) Cl Cl NH 3 Co NH 3 Cl Cl Co mer-triamminetrichlorocobalt(III)
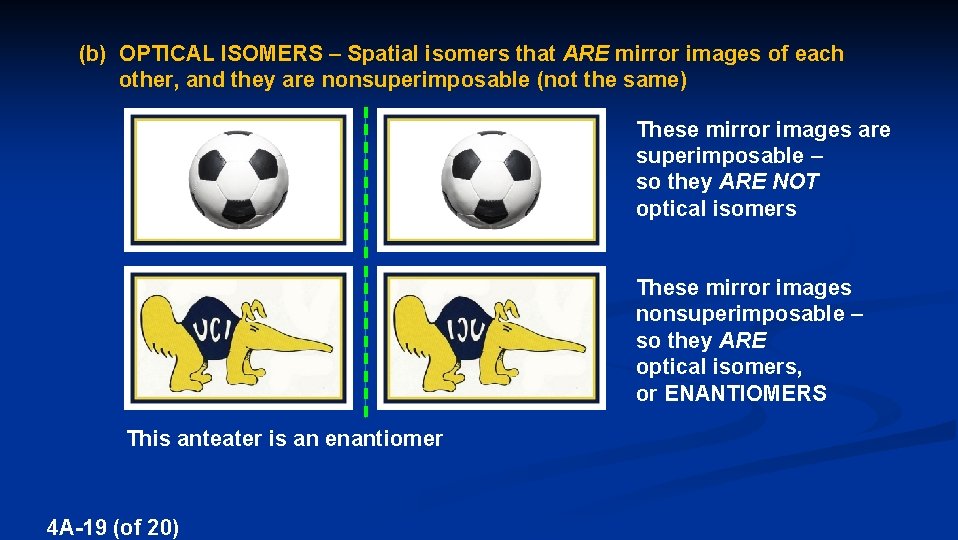
(b) OPTICAL ISOMERS – Spatial isomers that ARE mirror images of each other, and they are nonsuperimposable (not the same) These mirror images are superimposable – so they ARE NOT optical isomers These mirror images nonsuperimposable – so they ARE optical isomers, or ENANTIOMERS This anteater is an enantiomer 4 A-19 (of 20)
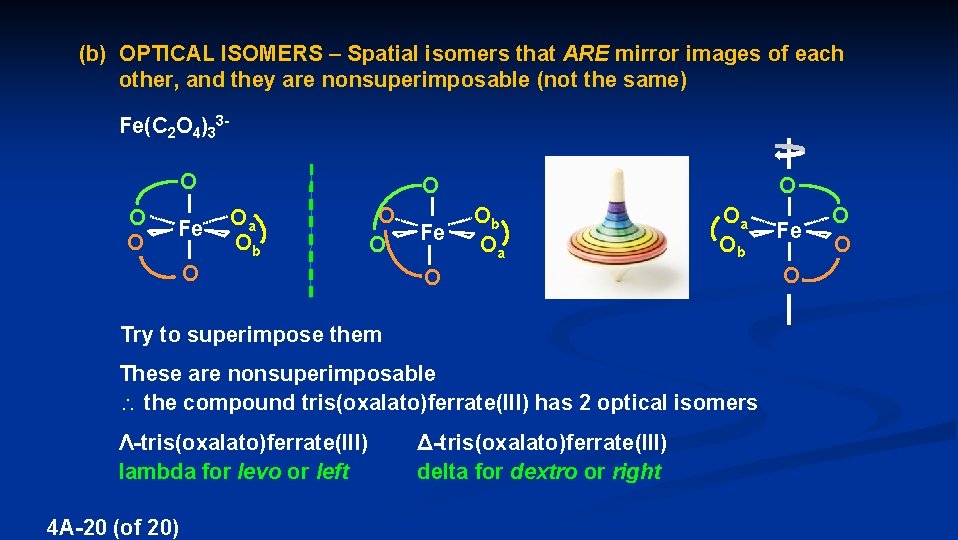
(b) OPTICAL ISOMERS – Spatial isomers that ARE mirror images of each other, and they are nonsuperimposable (not the same) Fe(C 2 O 4)33 O Oa Fe Ob O O O Fe O Try to superimpose them Ob Oa spin 180º O O Oa Fe Ob O These are nonsuperimposable the compound tris(oxalato)ferrate(III) has 2 optical isomers Ʌ-tris(oxalato)ferrate(III) lambda for levo or left 4 A-20 (of 20) Δ-tris(oxalato)ferrate(III) delta for dextro or right O O
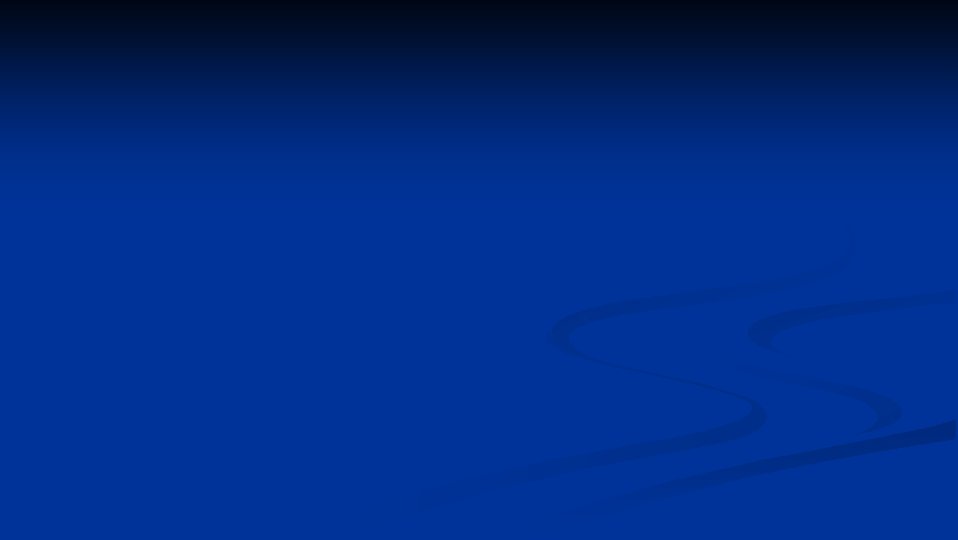
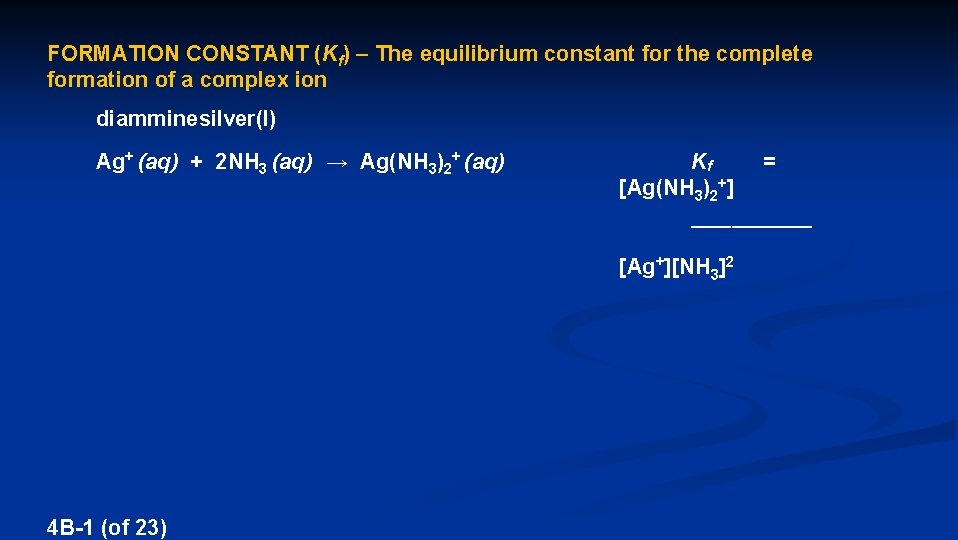
FORMATION CONSTANT (Kf) – The equilibrium constant for the complete formation of a complex ion diamminesilver(I) Ag+ (aq) + 2 NH 3 (aq) → Ag(NH 3)2+ (aq) Kf [Ag(NH 3)2+] = ________ [Ag+][NH 3]2 4 B-1 (of 23)
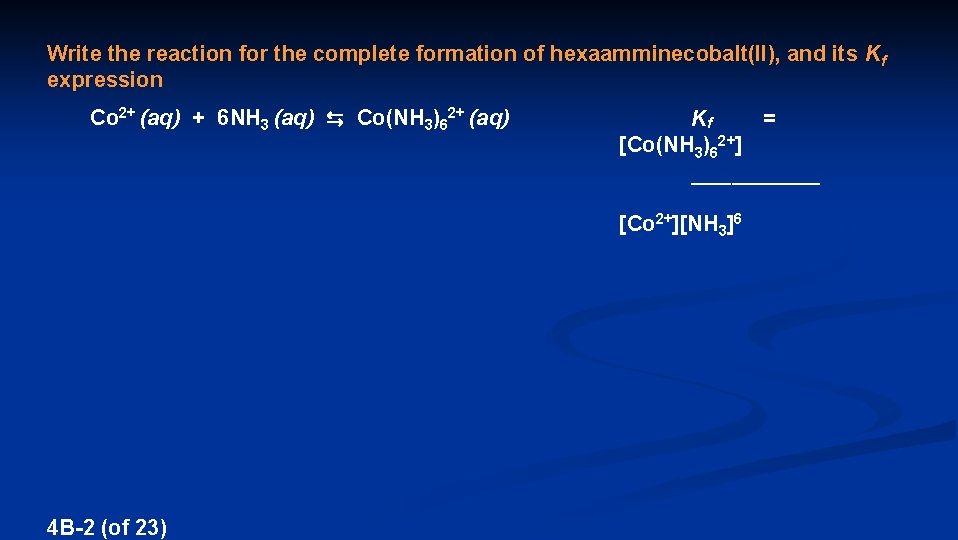
Write the reaction for the complete formation of hexaamminecobalt(II), and its Kf expression Co 2+ (aq) + 6 NH 3 (aq) ⇆ Co(NH 3)62+ (aq) Kf = [Co(NH 3)62+] ________ [Co 2+][NH 3]6 4 B-2 (of 23)
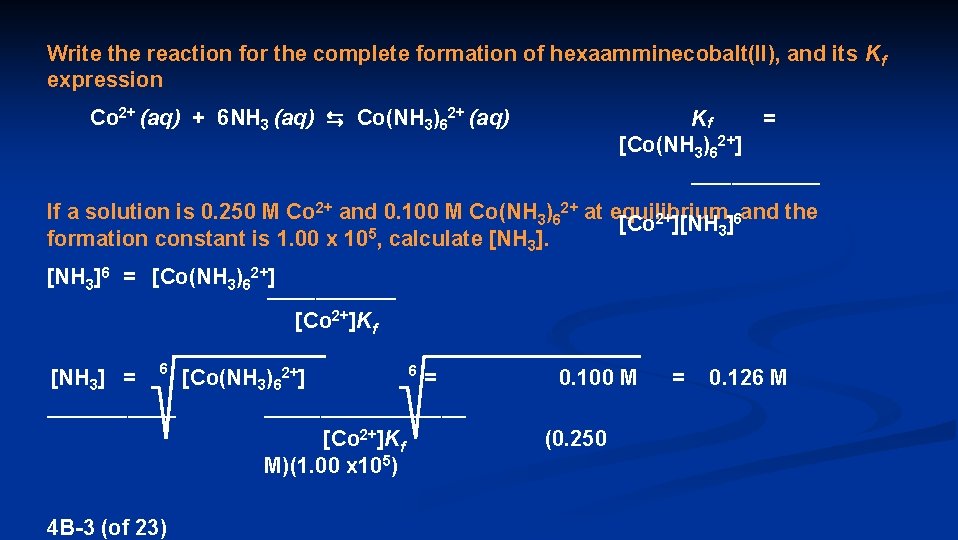
Write the reaction for the complete formation of hexaamminecobalt(II), and its Kf expression Co 2+ (aq) + 6 NH 3 (aq) ⇆ Co(NH 3)62+ (aq) Kf = [Co(NH 3)62+] ________ If a solution is 0. 250 M Co 2+ and 0. 100 M Co(NH 3)62+ at equilibrium, and the [Co 2+][NH 3]6 5 formation constant is 1. 00 x 10 , calculate [NH 3]6 = [Co(NH 3)62+] ________ [Co 2+]K f 6 2+ [NH 3] = [Co(NH 3 )6 ] ________ 6 = 0. 100 M _____________ [Co 2+]K M)(1. 00 x 105) 4 B-3 (of 23) f (0. 250 = 0. 126 M
![The formation constant for diamminesilverI is 1 00 x 106 Calculate Ag in a The formation constant for diamminesilver(I) is 1. 00 x 106. Calculate [Ag+] in a](https://slidetodoc.com/presentation_image_h/20ac91025ecf151ea91f5edf1dffa241/image-28.jpg)
The formation constant for diamminesilver(I) is 1. 00 x 106. Calculate [Ag+] in a solution that was originally 0. 100 M Ag+ and 0. 500 M NH 3. Ag+ (aq) + 2 NH 3 (aq) ⇆ Ag(NH 3)2+ (aq) Initial M’s Change in M’s Equilibrium M’s 0. 100 - x 0. 500 - 2 x 0 + x x The reaction is going in the forward direction and has a large equilibrium constant, x will be a large number 4 B-4 (of 23)
![The formation constant for diamminesilverI is 1 00 x 106 Calculate Ag in a The formation constant for diamminesilver(I) is 1. 00 x 106. Calculate [Ag+] in a](https://slidetodoc.com/presentation_image_h/20ac91025ecf151ea91f5edf1dffa241/image-29.jpg)
The formation constant for diamminesilver(I) is 1. 00 x 106. Calculate [Ag+] in a solution that was originally 0. 100 M Ag+ and 0. 500 M NH 3. Ag+ (aq) + 2 NH 3 (aq) ⇆ Ag(NH 3)2+ (aq) Initial M’s Shift M’s New Initial M’s Change M’s Equilibrium M’s 0. 100 - 0. 100 0 + x x Kf = [Ag(NH 3)2+] ________ [Ag+][NH 3] 0 + 0. 100 - x 1. 00 x 106 = (0. 100 – x) 2 x = 1. 11 x 10 -6 = [Ag+] 4 B-5 (of 23) 0. 500 - 0. 200 0. 300 + 2 x __________ (x)(0. 300 + 2 x)2 1. 00 x 106 = (0. 100) _______ (x)(0. 300)2
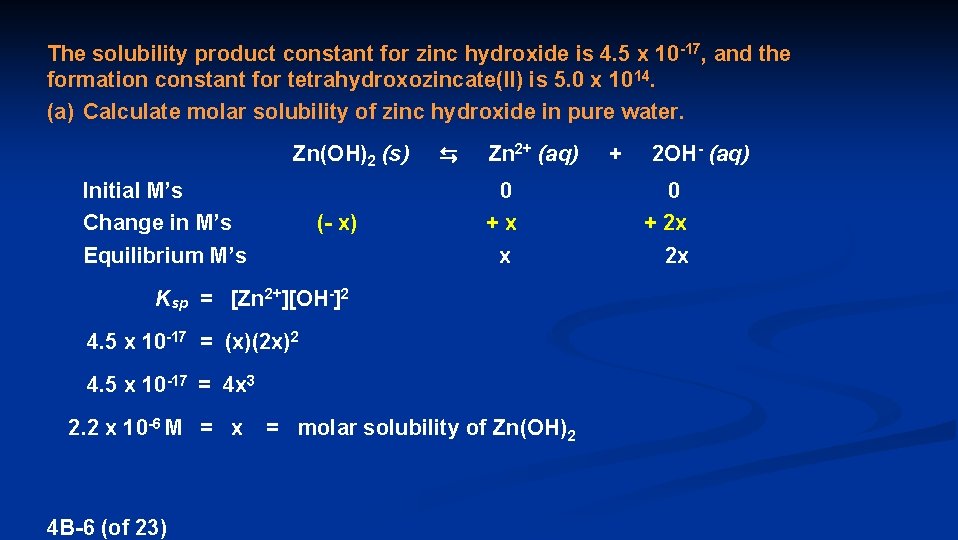
The solubility product constant for zinc hydroxide is 4. 5 x 10 -17, and the formation constant for tetrahydroxozincate(II) is 5. 0 x 1014. (a) Calculate molar solubility of zinc hydroxide in pure water. Zn(OH)2 (s) Initial M’s Change in M’s Equilibrium M’s (- x) ⇆ Zn 2+ (aq) + 2 OH- (aq) 0 + x x Ksp = [Zn 2+][OH-]2 4. 5 x 10 -17 = (x)(2 x)2 4. 5 x 10 -17 = 4 x 3 2. 2 x 10 -6 M = x = molar solubility of Zn(OH)2 4 B-6 (of 23) 0 + 2 x 2 x
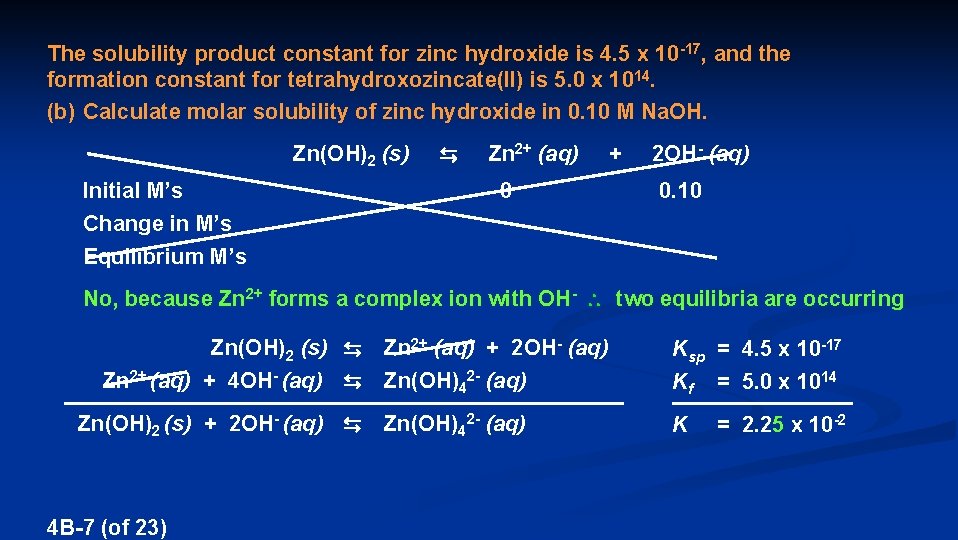
The solubility product constant for zinc hydroxide is 4. 5 x 10 -17, and the formation constant for tetrahydroxozincate(II) is 5. 0 x 1014. (b) Calculate molar solubility of zinc hydroxide in 0. 10 M Na. OH. Zn(OH)2 (s) Initial M’s Change in M’s Equilibrium M’s ⇆ Zn 2+ (aq) + 2 OH- (aq) 0 0. 10 No, because Zn 2+ forms a complex ion with OH- two equilibria are occurring Zn(OH)2 (s) ⇆ Zn 2+ (aq) + 2 OH- (aq) Zn 2+ (aq) + 4 OH- (aq) ⇆ Zn(OH)42 - (aq) Zn(OH)2 (s) + 2 OH- (aq) ⇆ Zn(OH)42 - (aq) 4 B-7 (of 23) Ksp = 4. 5 x 10 -17 Kf = 5. 0 x 1014 K = 2. 25 x 10 -2
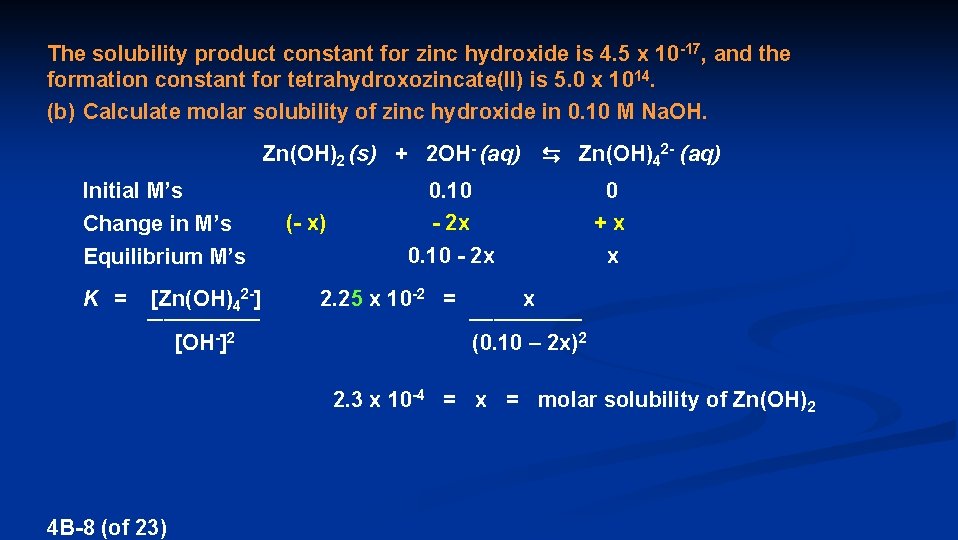
The solubility product constant for zinc hydroxide is 4. 5 x 10 -17, and the formation constant for tetrahydroxozincate(II) is 5. 0 x 1014. (b) Calculate molar solubility of zinc hydroxide in 0. 10 M Na. OH. Zn(OH)2 (s) + 2 OH- (aq) ⇆ Zn(OH)42 - (aq) Initial M’s Change in M’s Equilibrium M’s K = [Zn(OH)42 -] _______ [OH-]2 (- x) 0. 10 - 2 x 0 + x x 2. 25 x 10 -2 = x _______ (0. 10 – 2 x)2 2. 3 x 10 -4 = x = molar solubility of Zn(OH)2 4 B-8 (of 23)
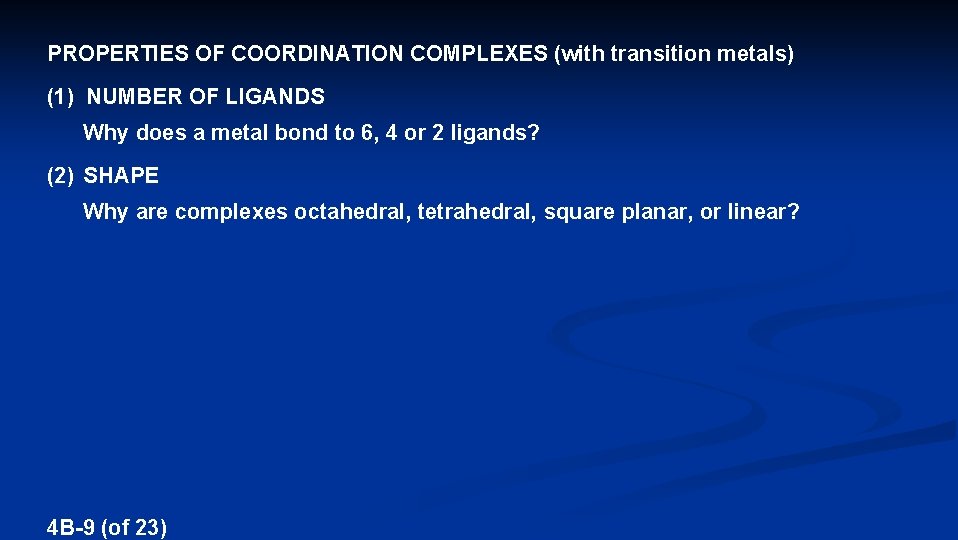
PROPERTIES OF COORDINATION COMPLEXES (with transition metals) (1) NUMBER OF LIGANDS Why does a metal bond to 6, 4 or 2 ligands? (2) SHAPE Why are complexes octahedral, tetrahedral, square planar, or linear? 4 B-9 (of 23)
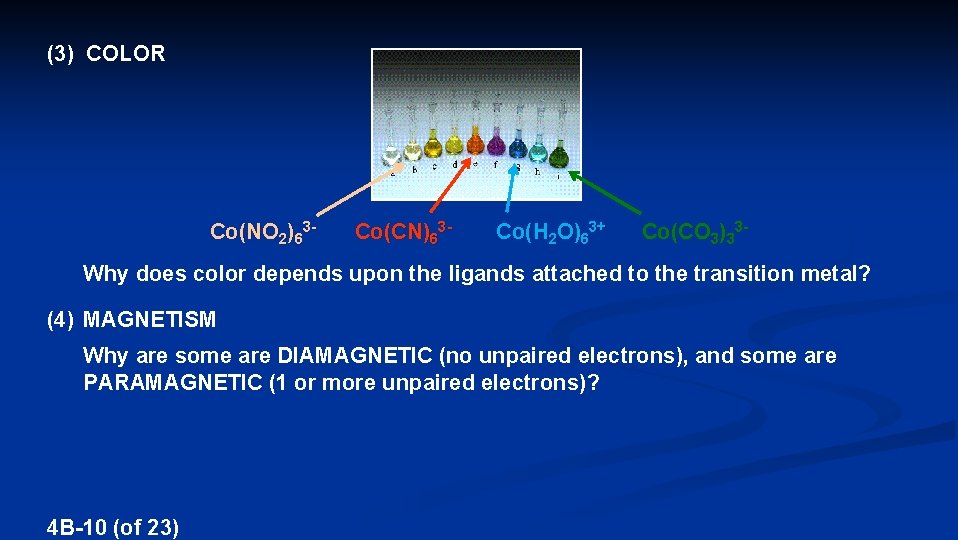
(3) COLOR Co(NO 2)63 - Co(CN)63 - Co(H 2 O)63+ Co(CO 3)33 - Why does color depends upon the ligands attached to the transition metal? (4) MAGNETISM Why are some are DIAMAGNETIC (no unpaired electrons), and some are PARAMAGNETIC (1 or more unpaired electrons)? 4 B-10 (of 23)
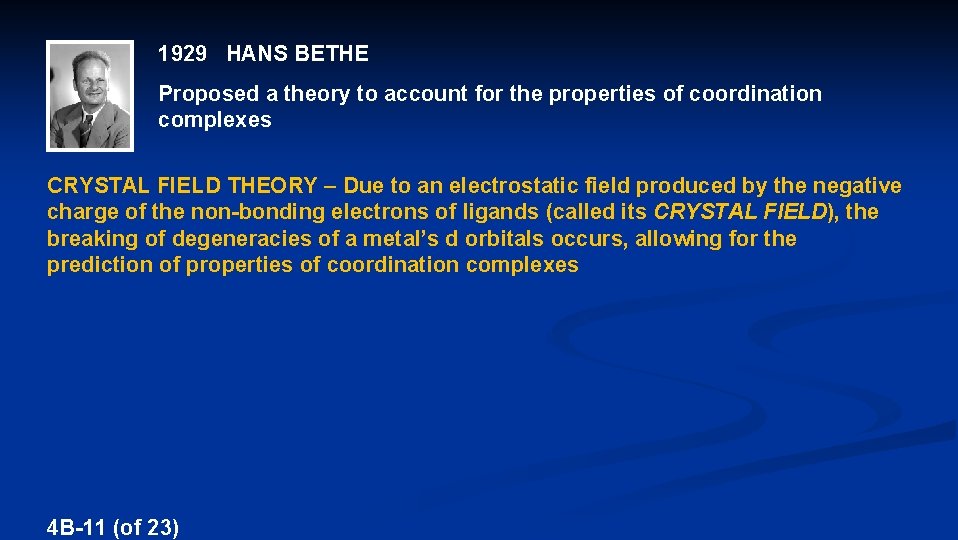
1929 HANS BETHE Proposed a theory to account for the properties of coordination complexes CRYSTAL FIELD THEORY – Due to an electrostatic field produced by the negative charge of the non-bonding electrons of ligands (called its CRYSTAL FIELD), the breaking of degeneracies of a metal’s d orbitals occurs, allowing for the prediction of properties of coordination complexes 4 B-11 (of 23)
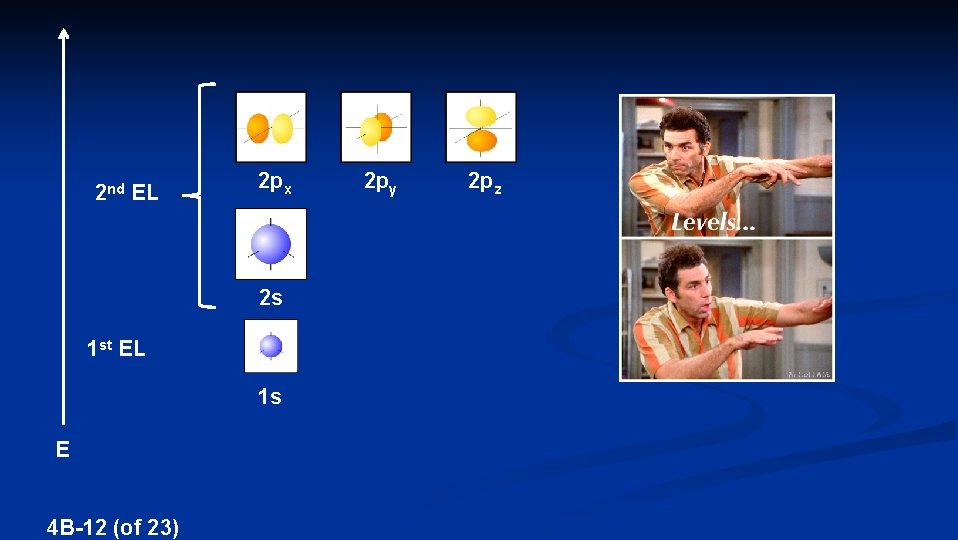
2 nd EL 2 px 2 s 1 st EL 1 s E 4 B-12 (of 23) 2 py 2 pz
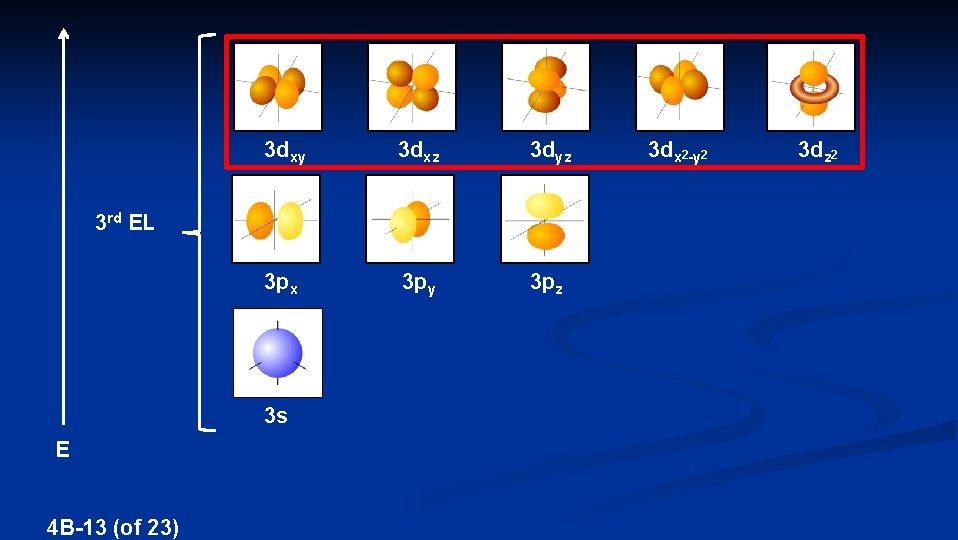
3 dxy 3 dxz 3 dyz 3 px 3 py 3 pz 3 rd EL 3 s E 4 B-13 (of 23) 3 dx 2 -y 2 3 dz 2
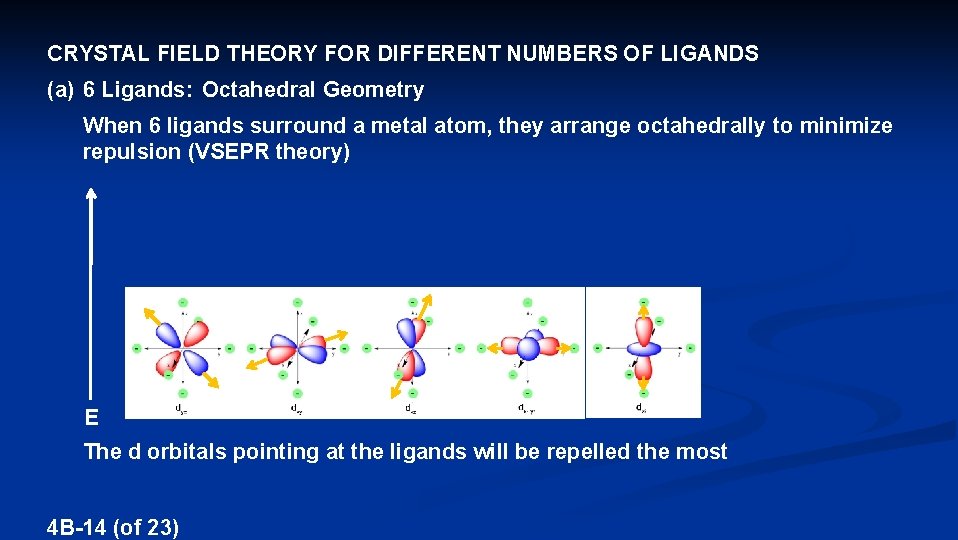
CRYSTAL FIELD THEORY FOR DIFFERENT NUMBERS OF LIGANDS (a) 6 Ligands: Octahedral Geometry When 6 ligands surround a metal atom, they arrange octahedrally to minimize repulsion (VSEPR theory) E The d orbitals pointing at the ligands will be repelled the most 4 B-14 (of 23)
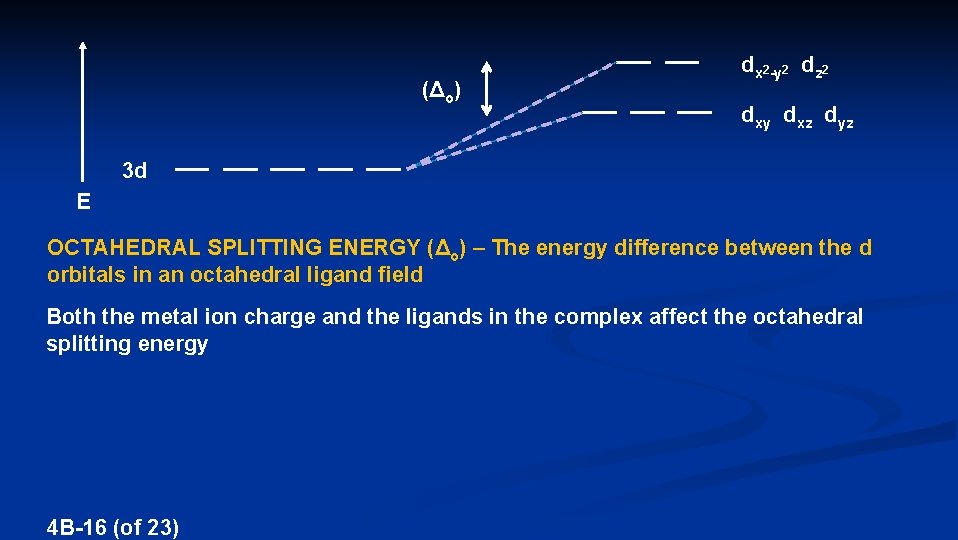
(Δo) dx 2 -y 2 dz 2 dxy dxz dyz 3 d E OCTAHEDRAL SPLITTING ENERGY (Δo) – The energy difference between the d orbitals in an octahedral ligand field Both the metal ion charge and the ligands in the complex affect the octahedral splitting energy 4 B-16 (of 23)
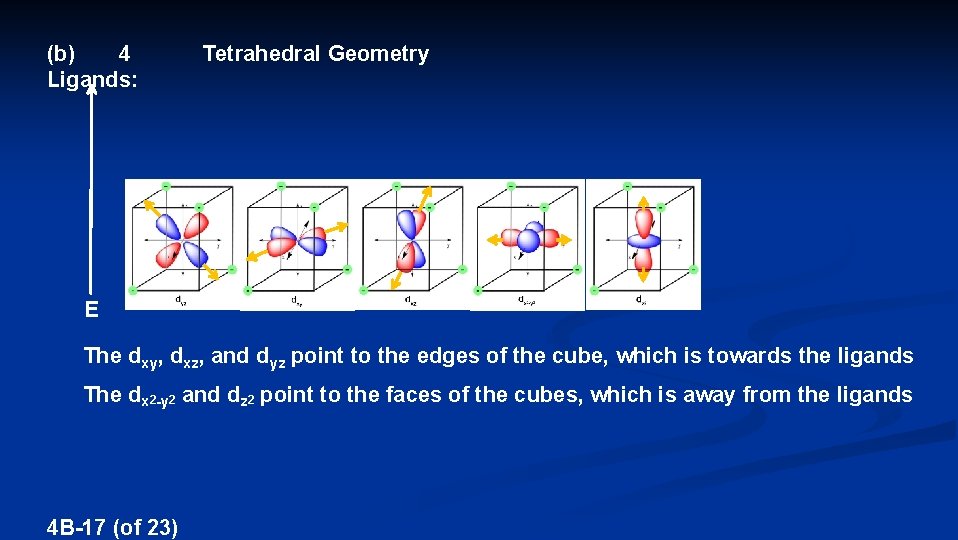
(b) 4 Ligands: Tetrahedral Geometry E The dxy, dxz, and dyz point to the edges of the cube, which is towards the ligands The dx 2 -y 2 and dz 2 point to the faces of the cubes, which is away from the ligands 4 B-17 (of 23)
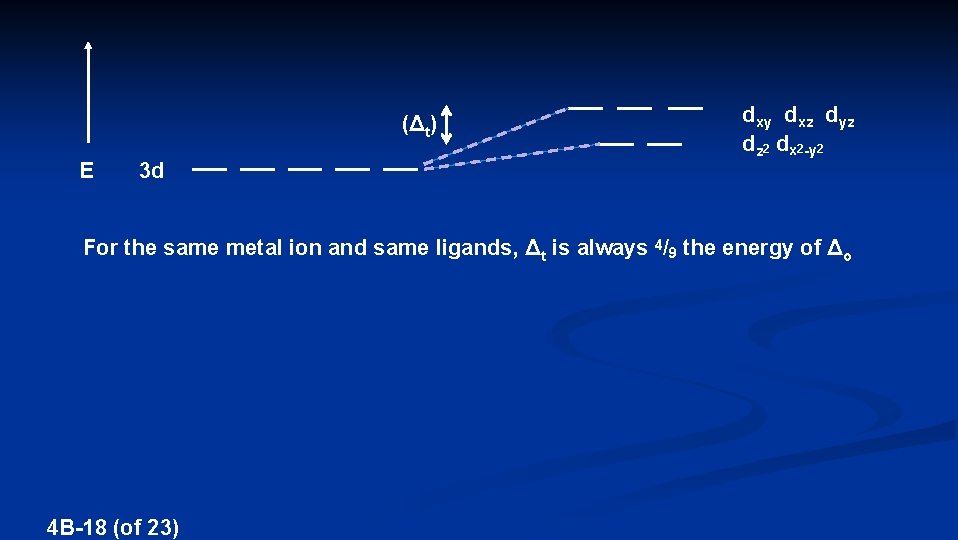
(Δt) E 3 d dxy dxz dyz dz 2 dx 2 -y 2 For the same metal ion and same ligands, Δt is always 4/9 the energy of Δo 4 B-18 (of 23)
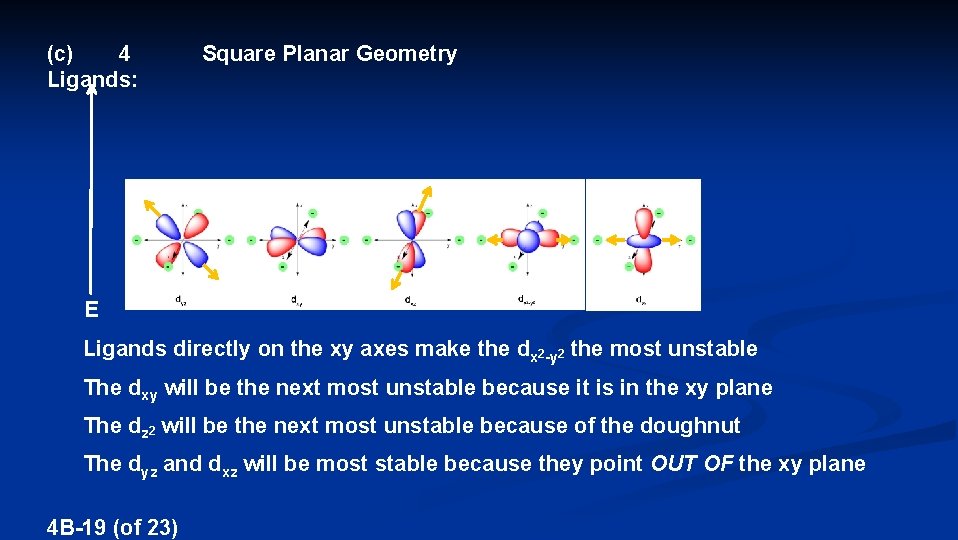
(c) 4 Ligands: Square Planar Geometry E Ligands directly on the xy axes make the dx 2 -y 2 the most unstable The dxy will be the next most unstable because it is in the xy plane The dz 2 will be the next most unstable because of the doughnut The dyz and dxz will be most stable because they point OUT OF the xy plane 4 B-19 (of 23)
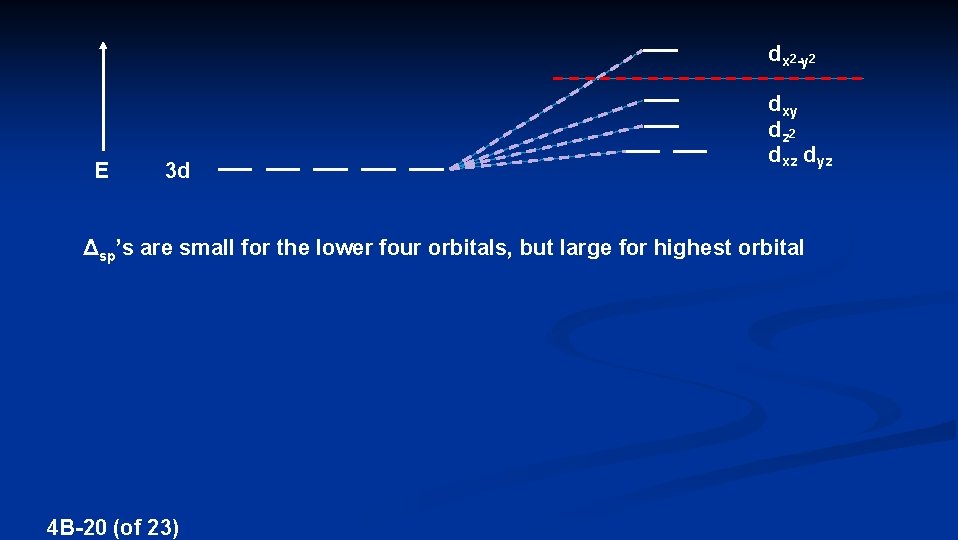
dx 2 -y 2 E 3 d dxy dz 2 dxz dyz Δsp’s are small for the lower four orbitals, but large for highest orbital 4 B-20 (of 23)
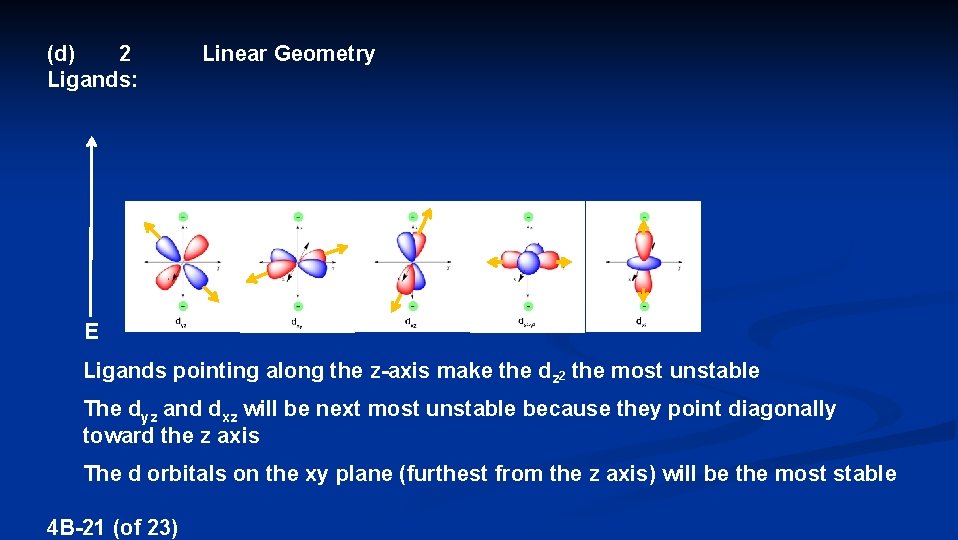
(d) 2 Ligands: Linear Geometry E Ligands pointing along the z-axis make the dz 2 the most unstable The dyz and dxz will be next most unstable because they point diagonally toward the z axis The d orbitals on the xy plane (furthest from the z axis) will be the most stable 4 B-21 (of 23)
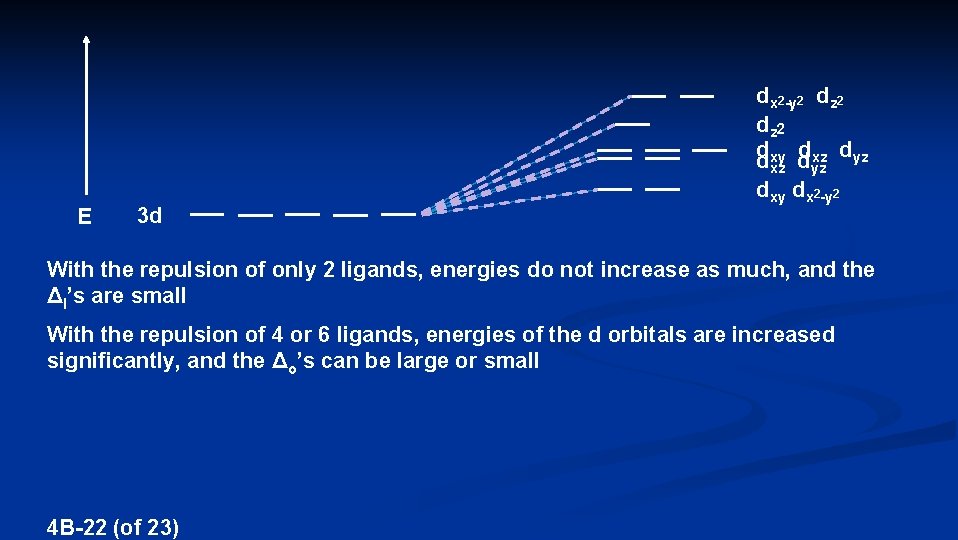
dx 2 -y 2 dz 2 d dxz dyz dxy d xz E 3 d yz dxy dx 2 -y 2 With the repulsion of only 2 ligands, energies do not increase as much, and the Δl’s are small With the repulsion of 4 or 6 ligands, energies of the d orbitals are increased significantly, and the Δo’s can be large or small 4 B-22 (of 23)
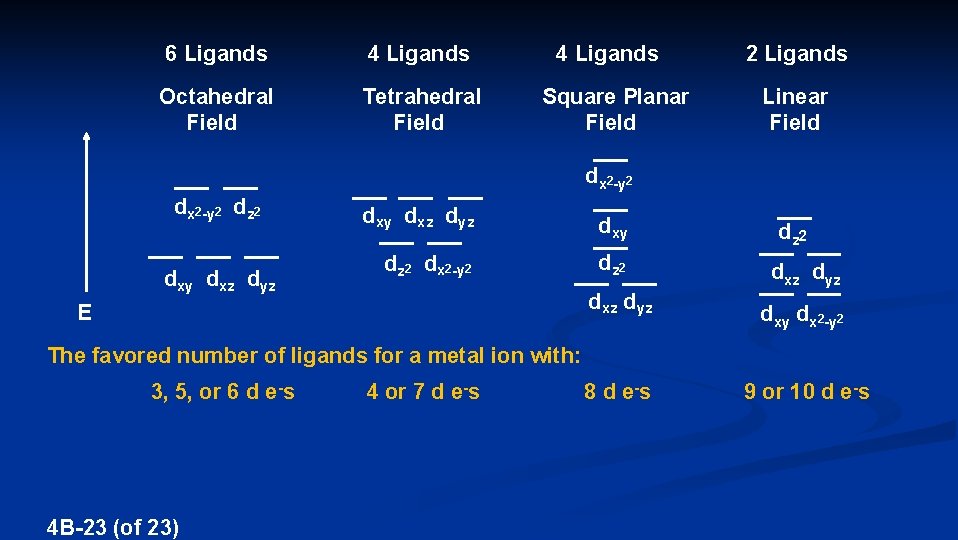
6 Ligands 4 Ligands Octahedral Field Tetrahedral Field 4 Ligands Square Planar Field 2 Ligands Linear Field dx 2 -y 2 dz 2 dxy dxz dyz dxy dz 2 dx 2 -y 2 dz 2 dxz dyz E dz 2 dxz dyz dxy dx 2 -y 2 The favored number of ligands for a metal ion with: 3, 5, or 6 d e-s 4 B-23 (of 23) 4 or 7 d e-s 8 d e-s 9 or 10 d e-s
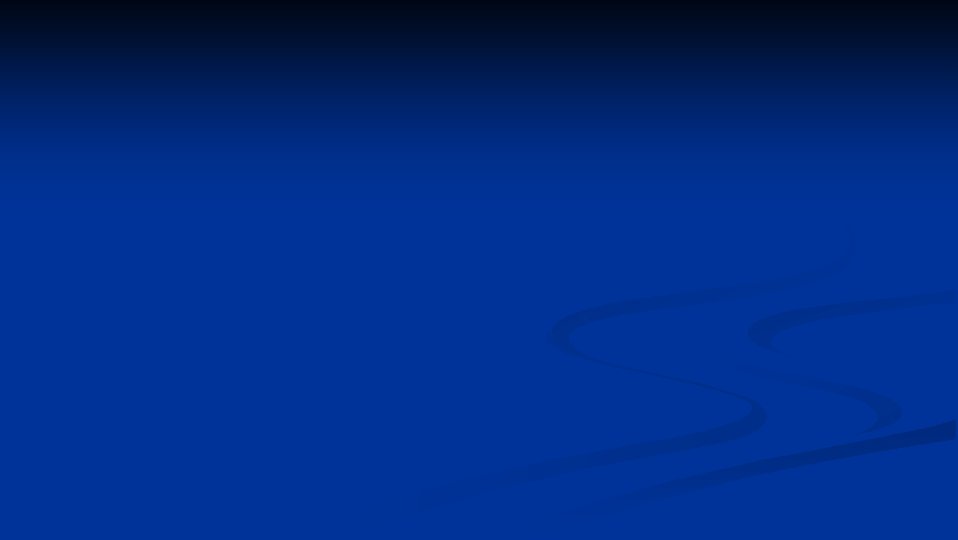
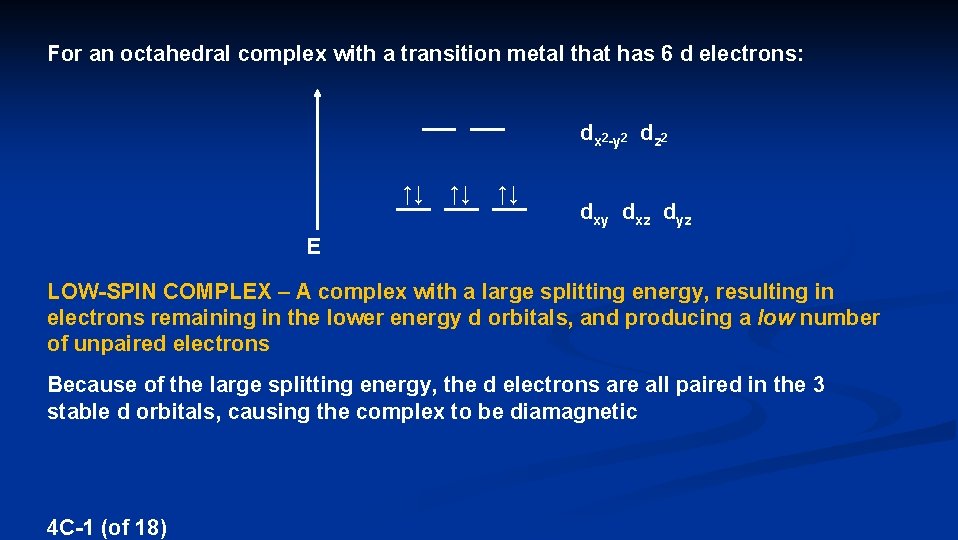
For an octahedral complex with a transition metal that has 6 d electrons: dx 2 -y 2 dz 2 ↑↓ ↑↓ ↑↓ dxy dxz dyz E LOW-SPIN COMPLEX – A complex with a large splitting energy, resulting in electrons remaining in the lower energy d orbitals, and producing a low number of unpaired electrons Because of the large splitting energy, the d electrons are all paired in the 3 stable d orbitals, causing the complex to be diamagnetic 4 C-1 (of 18)
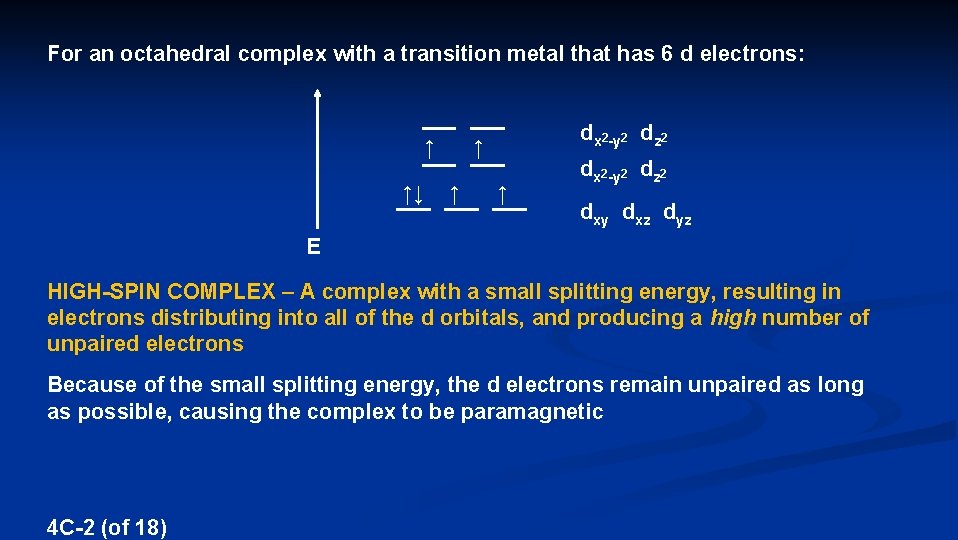
For an octahedral complex with a transition metal that has 6 d electrons: ↑ ↑↓ dx 2 -y 2 dz 2 ↑ ↑ ↑ dx 2 -y 2 dz 2 dxy dxz dyz E HIGH-SPIN COMPLEX – A complex with a small splitting energy, resulting in electrons distributing into all of the d orbitals, and producing a high number of unpaired electrons Because of the small splitting energy, the d electrons remain unpaired as long as possible, causing the complex to be paramagnetic 4 C-2 (of 18)
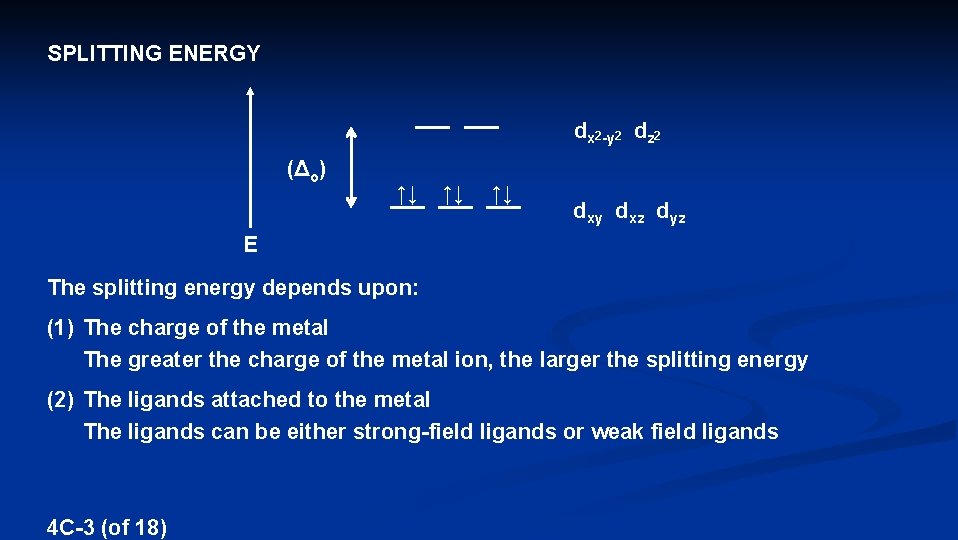
SPLITTING ENERGY dx 2 -y 2 dz 2 (Δo) ↑↓ ↑↓ ↑↓ dxy dxz dyz E The splitting energy depends upon: (1) The charge of the metal The greater the charge of the metal ion, the larger the splitting energy (2) The ligands attached to the metal The ligands can be either strong-field ligands or weak field ligands 4 C-3 (of 18)
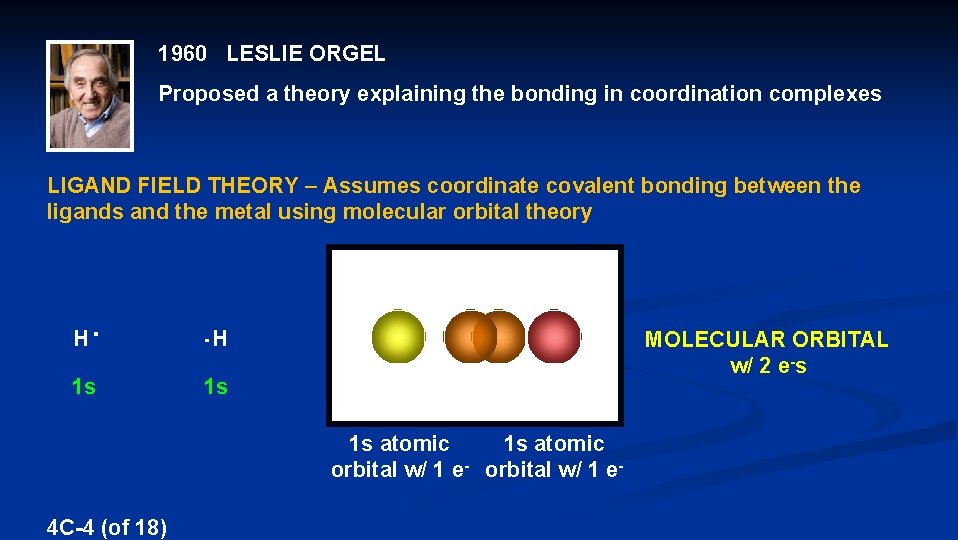
1960 LESLIE ORGEL Proposed a theory explaining the bonding in coordination complexes LIGAND FIELD THEORY – Assumes coordinate covalent bonding between the ligands and the metal using molecular orbital theory H H 1 s 1 s MOLECULAR ORBITAL w/ 2 e-s 1 s atomic orbital w/ 1 e- 4 C-4 (of 18)
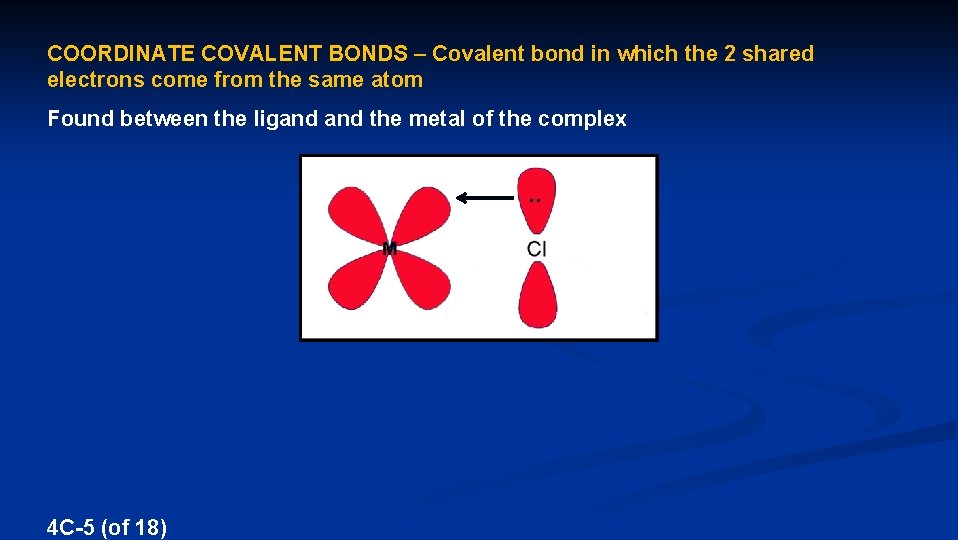
COORDINATE COVALENT BONDS – Covalent bond in which the 2 shared electrons come from the same atom Found between the ligand the metal of the complex . . 4 C-5 (of 18)
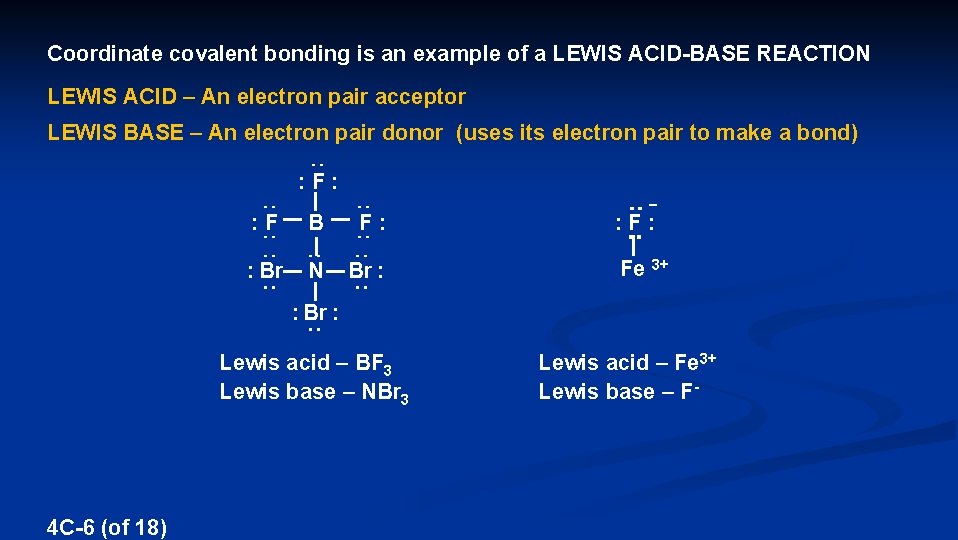
Coordinate covalent bonding is an example of a LEWIS ACID-BASE REACTION LEWIS ACID – An electron pair acceptor LEWIS BASE – An electron pair donor (uses its electron pair to make a bond) : F : – : F B F : : Br N Br : Fe 3+ : Br : Lewis acid – BF 3 Lewis base – NBr 3 4 C-6 (of 18) Lewis acid – Fe 3+ Lewis base – F-
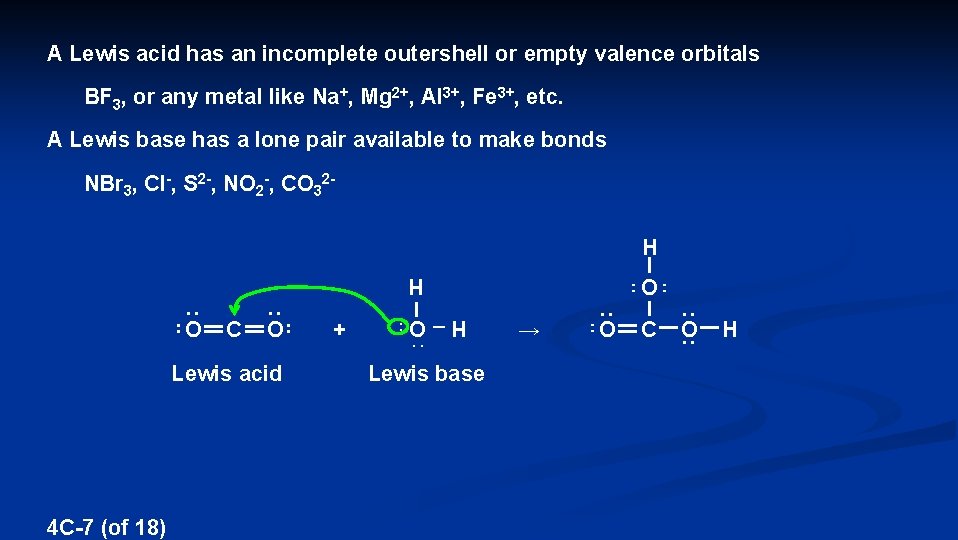
A Lewis acid has an incomplete outershell or empty valence orbitals BF 3, or any metal like Na+, Mg 2+, Al 3+, Fe 3+, etc. A Lewis base has a lone pair available to make bonds NBr 3, Cl-, S 2 -, NO 2 -, CO 32 H H Lewis acid 4 C-7 (of 18) + . . H – O : O C O Lewis base O → O C O H
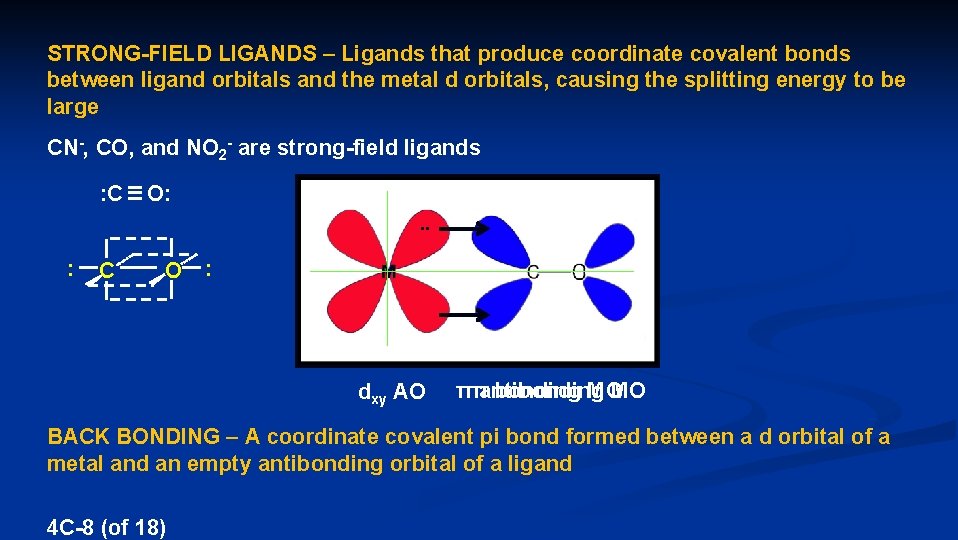
STRONG-FIELD LIGANDS – Ligands that produce coordinate covalent bonds between ligand orbitals and the metal d orbitals, causing the splitting energy to be large CN-, CO, and NO 2 - are strong-field ligands : C O: 2 pz. . : C O : sp sp 2 py dxy AO π antibonding MO π bonding MO BACK BONDING – A coordinate covalent pi bond formed between a d orbital of a metal and an empty antibonding orbital of a ligand 4 C-8 (of 18)
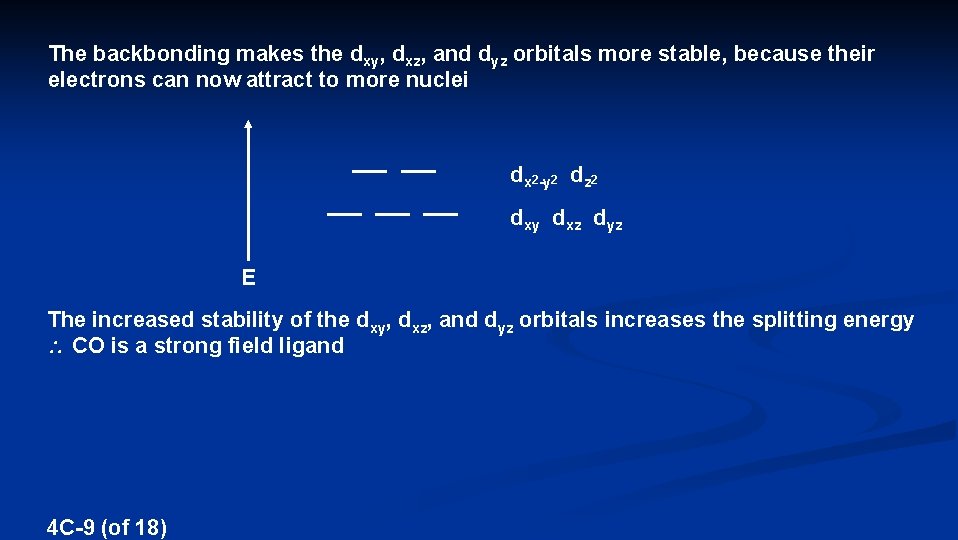
The backbonding makes the dxy, dxz, and dyz orbitals more stable, because their electrons can now attract to more nuclei dx 2 -y 2 dz 2 dxy dxz dyz E The increased stability of the dxy, dxz, and dyz orbitals increases the splitting energy CO is a strong field ligand 4 C-9 (of 18)
![FeCO64 4 C10 of 18 hexacarbonylferrateII Fe 2 Ar3 d 6 Fe(CO)64 - 4 C-10 (of 18) hexacarbonylferrate(II) Fe 2+: [Ar]3 d 6](https://slidetodoc.com/presentation_image_h/20ac91025ecf151ea91f5edf1dffa241/image-57.jpg)
Fe(CO)64 - 4 C-10 (of 18) hexacarbonylferrate(II) Fe 2+: [Ar]3 d 6
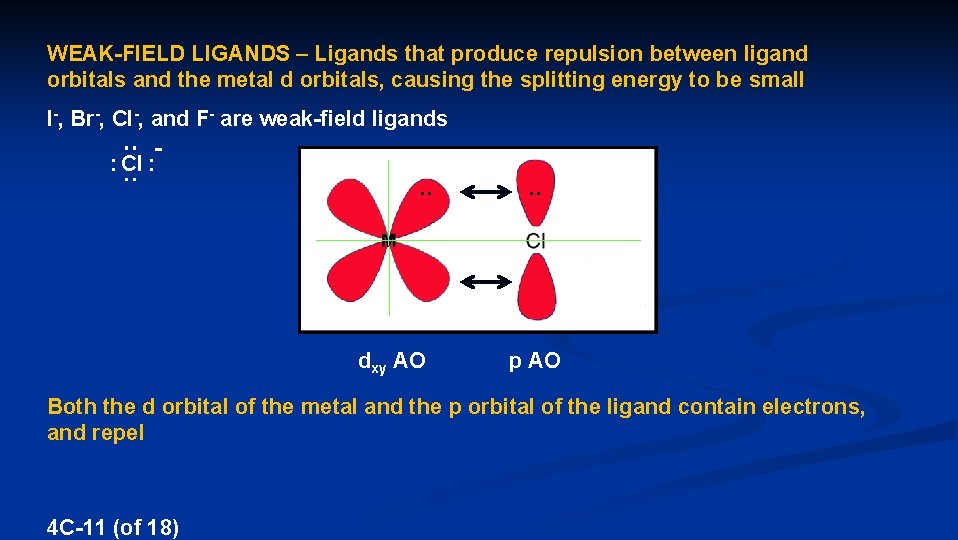
WEAK-FIELD LIGANDS – Ligands that produce repulsion between ligand orbitals and the metal d orbitals, causing the splitting energy to be small I-, Br-, Cl-, and F- are weak-field ligands : Cl : . . dxy AO . . p AO Both the d orbital of the metal and the p orbital of the ligand contain electrons, and repel 4 C-11 (of 18)
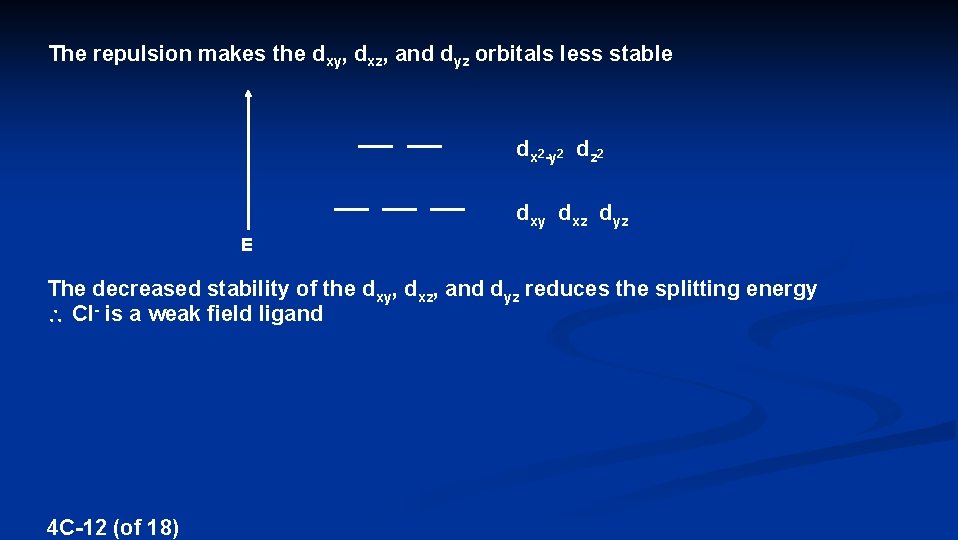
The repulsion makes the dxy, dxz, and dyz orbitals less stable dx 2 -y 2 dz 2 dxy dxz dyz E The decreased stability of the dxy, dxz, and dyz reduces the splitting energy Cl- is a weak field ligand 4 C-12 (of 18)
![Fe Cl 64 4 C13 of 18 hexachloroferrateII Fe 2 Ar3 d 6 Fe. Cl 64 - 4 C-13 (of 18) hexachloroferrate(II) Fe 2+: [Ar]3 d 6](https://slidetodoc.com/presentation_image_h/20ac91025ecf151ea91f5edf1dffa241/image-60.jpg)
Fe. Cl 64 - 4 C-13 (of 18) hexachloroferrate(II) Fe 2+: [Ar]3 d 6
![HexafluorocobaltateIII a Give the electron configuration of the cobalt ion Co atom Ar4 s Hexafluorocobaltate(III) (a) Give the electron configuration of the cobalt ion Co atom: [Ar]4 s](https://slidetodoc.com/presentation_image_h/20ac91025ecf151ea91f5edf1dffa241/image-61.jpg)
Hexafluorocobaltate(III) (a) Give the electron configuration of the cobalt ion Co atom: [Ar]4 s 23 d 7 Co 3+ ion: [Ar]3 d 6 (b) Identify the ligands as strong-field or weak-field (c) Draw the splitting pattern for the cobalt ↑ ↑↓ ↑ ↑ dx 2 -y 2 dz 2 ↑ dxy dxz dyz E (d) Identify the complex as high-spin or low-spin high-spin (e) Identify the complex as paramagnetic or diamagnetic paramagnetic 4 C-14 (of 18)
![HexacarbonylironII a Give the electron configuration of the iron ion Fe atom Ar4 s Hexacarbonyliron(II) (a) Give the electron configuration of the iron ion Fe atom: [Ar]4 s](https://slidetodoc.com/presentation_image_h/20ac91025ecf151ea91f5edf1dffa241/image-62.jpg)
Hexacarbonyliron(II) (a) Give the electron configuration of the iron ion Fe atom: [Ar]4 s 23 d 6 Fe 2+ ion: [Ar]3 d 6 (b) Identify the ligands as strong-field or weak-field strong-field (c) Draw the splitting pattern for the iron dx 2 -y 2 dz 2 ↑↓ E ↑↓ ↑↓ dxy dxz dyz (d) Identify the complex as high-spin or low-spin (e) Identify the complex as paramagnetic or diamagnetic 4 C-15 (of 18)
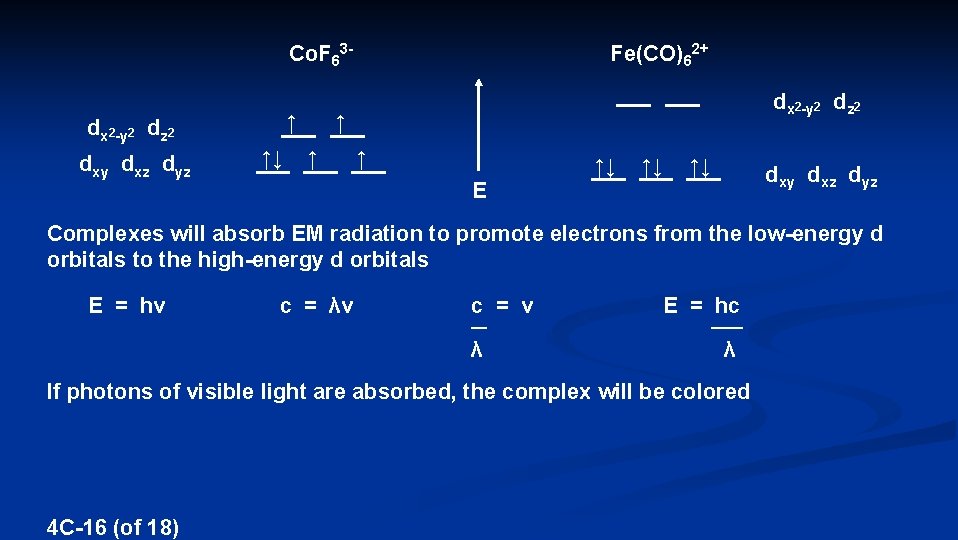
Co. F 63↑ dx 2 -y 2 dz 2 dxy dxz dyz ↑↓ Fe(CO)62+ dx 2 -y 2 dz 2 ↑ ↑↓ ↑↓ ↑↓ E dxy dxz dyz Complexes will absorb EM radiation to promote electrons from the low-energy d orbitals to the high-energy d orbitals E = hν c = λν c = ν E = hc λ λ __ ____ If photons of visible light are absorbed, the complex will be colored 4 C-16 (of 18)
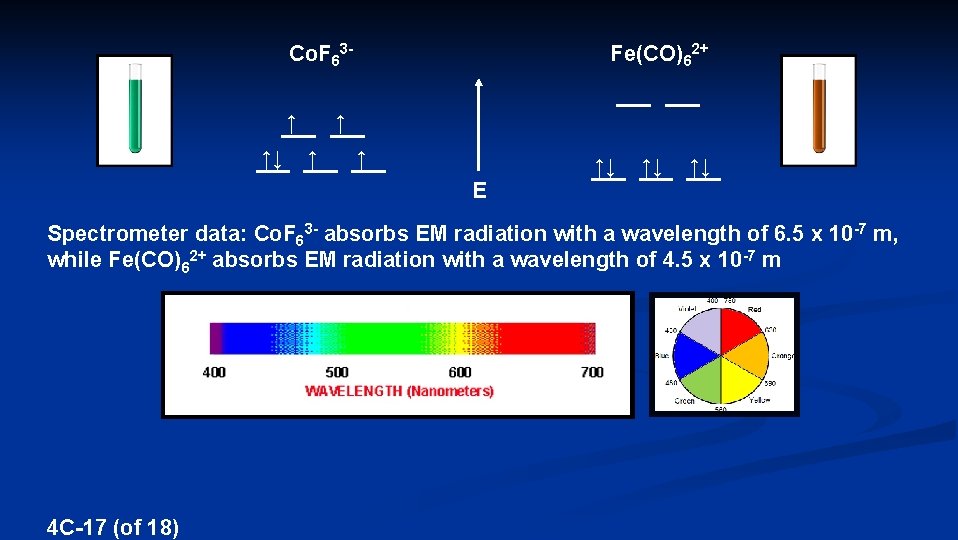
Co. F 63↑ ↑↓ Fe(CO)62+ ↑ ↑↓ ↑↓ ↑↓ E Spectrometer data: Co. F 63 - absorbs EM radiation with a wavelength of 6. 5 x 10 -7 m, while Fe(CO)62+ absorbs EM radiation with a wavelength of 4. 5 x 10 -7 m 4 C-17 (of 18)
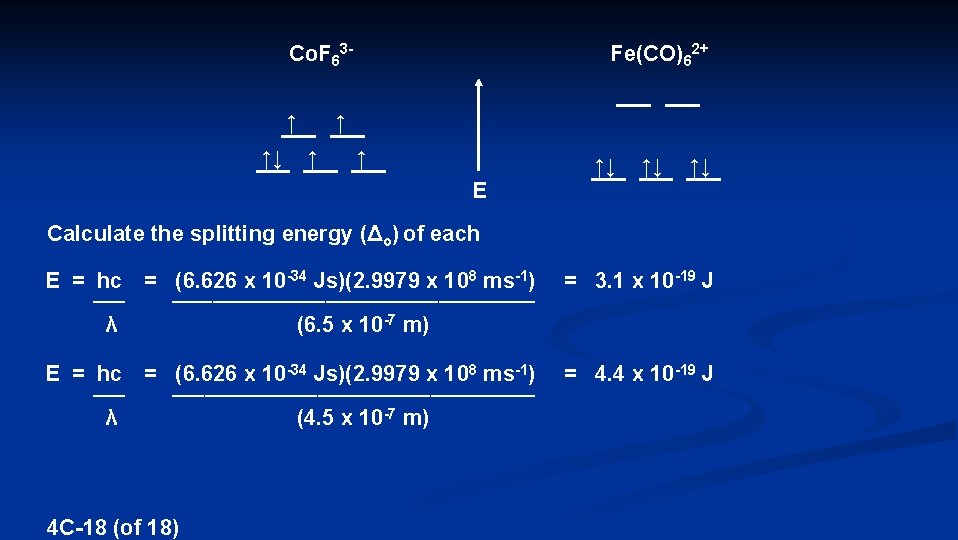
Co. F 63↑ ↑↓ Fe(CO)62+ ↑ ↑↓ ↑↓ ↑↓ E Calculate the splitting energy (Δo) of each E = hc = (6. 626 x 10 -34 Js)(2. 9979 x 108 ms-1) _________________________ λ (6. 5 x 10 -7 m) E = hc = (6. 626 x 10 -34 Js)(2. 9979 x 108 ms-1) _________________________ λ (4. 5 x 10 -7 m) 4 C-18 (of 18) = 3. 1 x 10 -19 J = 4. 4 x 10 -19 J
Ejemplo de fuerza ion ion
Qumica
Fuerzas dipolo dipolo ejemplos
What is london forces
M(ab)3 isomers
Hexaaquaaluminium complex ion
Solubility and complex ion equilibria
Complex ions naming
A linear complex ion with ligands on the x-axis
A linear complex ion with ligands on the x-axis
Monatomic ion definition chemistry
Polyatomic ion sheet
Charges of compounds
Monatomic ion definition chemistry
Tetrahedral coordination complex
There aren't some cakes
Any to any connectivity
Any question atau any questions
Electronic spectra of coordination compounds
Correlation diagram in coordination chemistry
Coordination number
Bruno dislikes sitting on the beach
Ghon complex and ranke complex
Quiz on compound sentences
Electra complex vs oedipus complex
Difference between oedipus complex and electra complex
Mbti breakdown
Ib organic chemistry
Inorganic chemistry vs organic chemistry
Italian gains in ww1
Is kno3 acidic basic or neutral
Fats and lipids
Thermoneutral environment for neonates
There is no neutral ground in the universe
Delta neutral hedging in surat
Condicionamiento clasico y operante
Lexical twins
Sense in semantics
Gender-neutral housing pros and cons
Stuck in neutral summary
Put price formula
Neutral mutation
Unconditioned stimulus unconditioned response
Neutral fat
Associative learning
Translation by a more general word examples
Java ul
Talk verb 1 2 3
What are some neutral mutations
Neutral mutation
Neutral fat
Neutral ferric chloride test
Electrostatic forces meaning
Types of dictions
Choice of diction
What is formal diction examples
Shy connotation
Triamminetriaquachromium(iii)
Estimulo neutral
Amino acid r groups
Definition of higher order conditioning
Site:slidetodoc.com
Good news and neutral messages
Neutral theory of molecular evolution notes
Risk neutral probability formula
Binomial tree with dividend