Chemistry 163 General Chemistry 3 Chapter 17 Electrochemistry
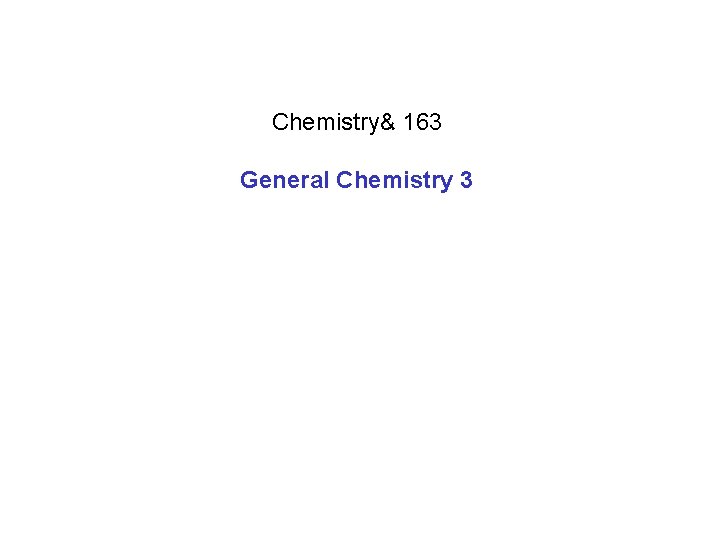
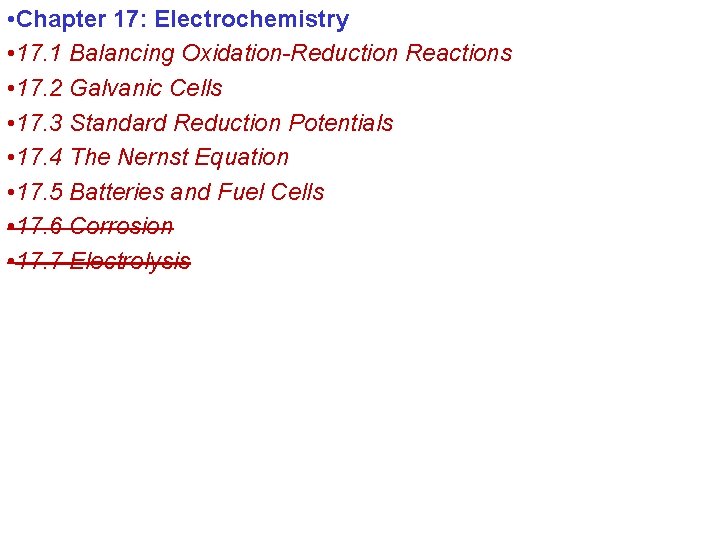
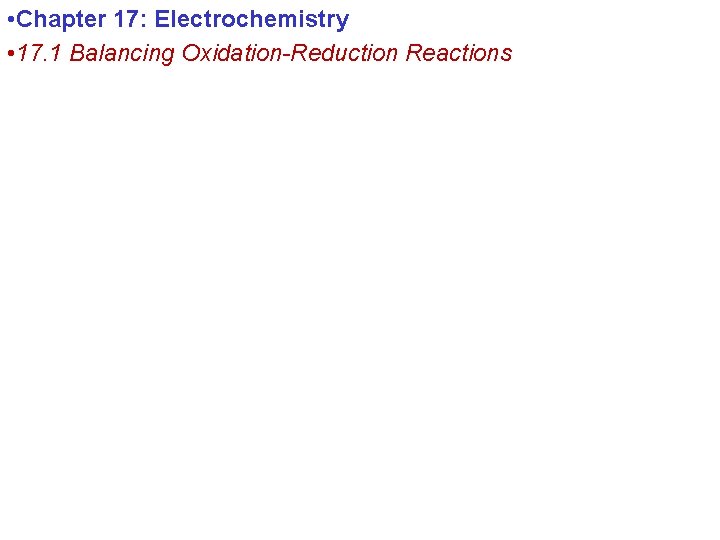
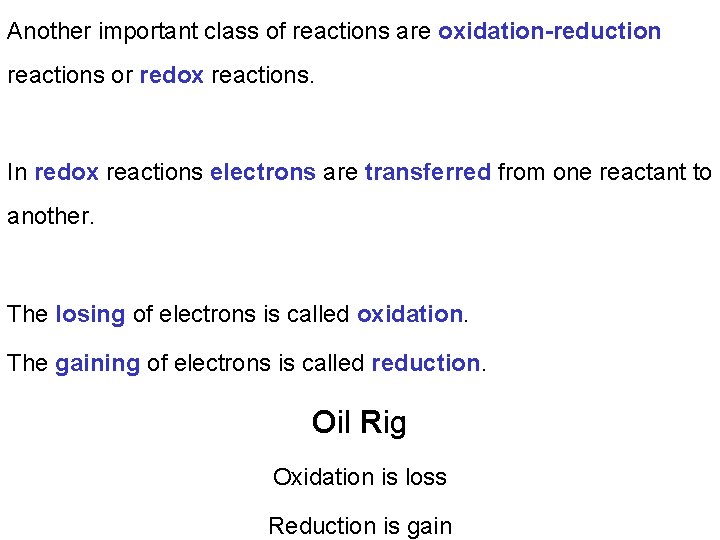
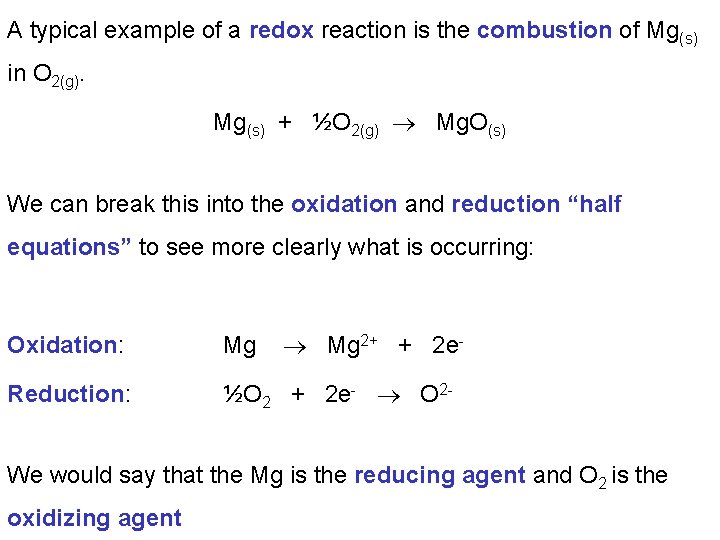
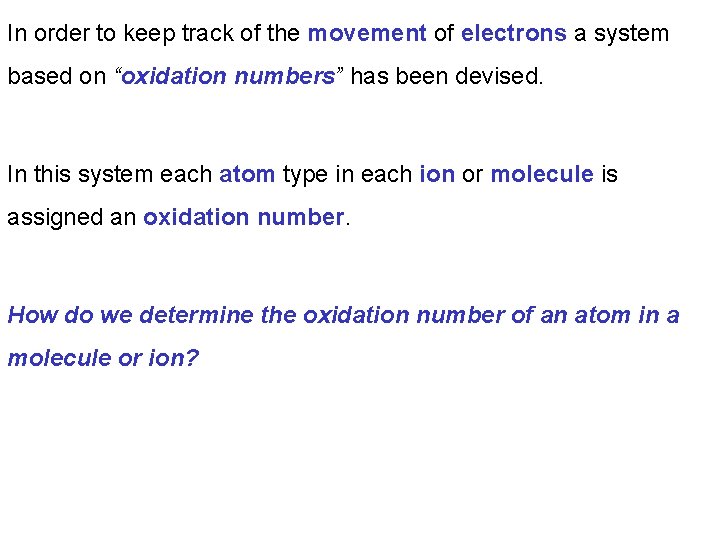
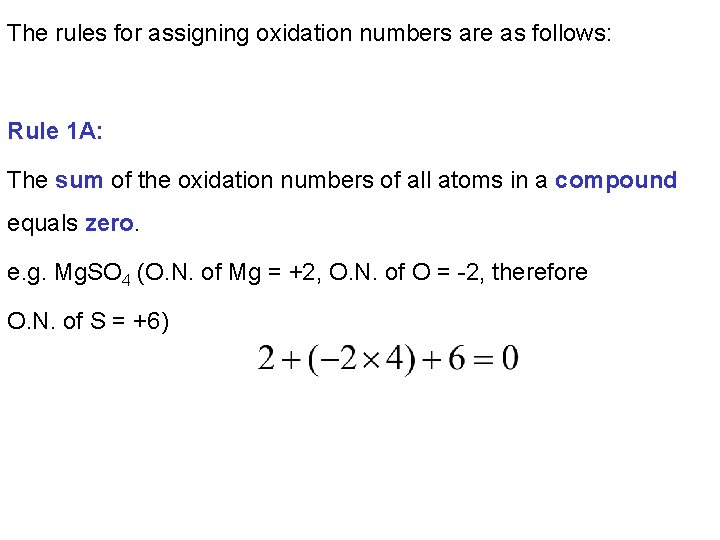
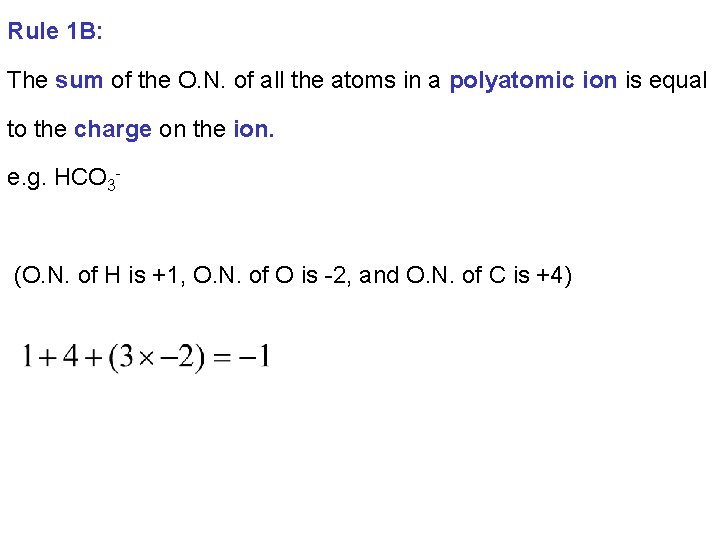
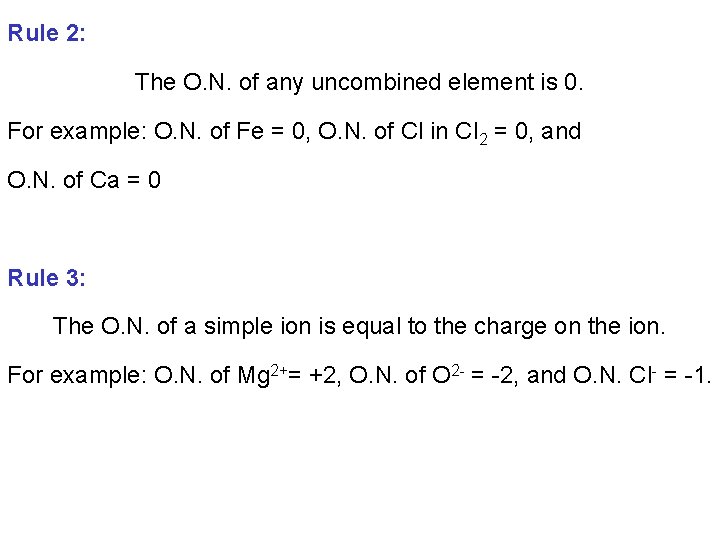
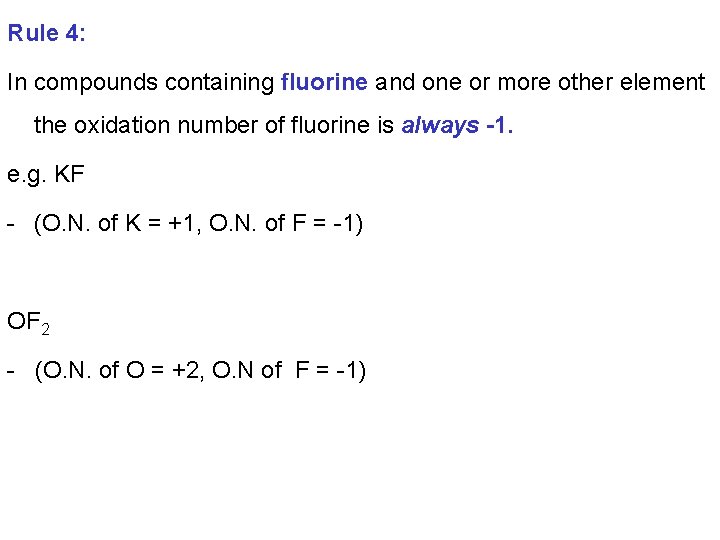
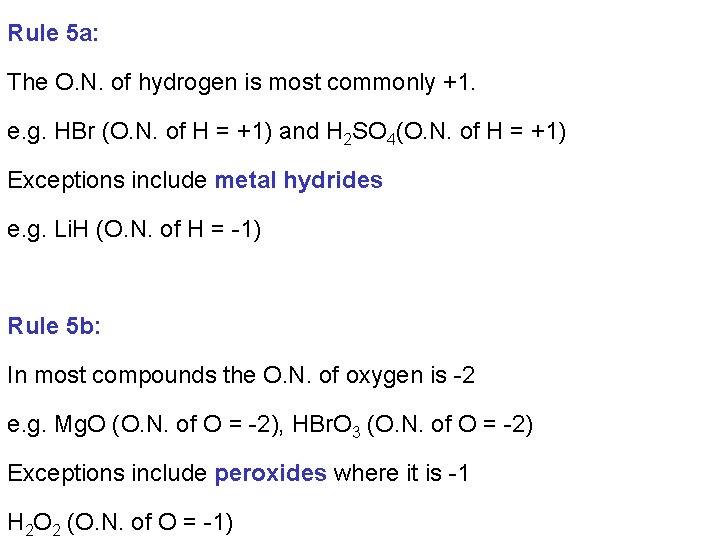
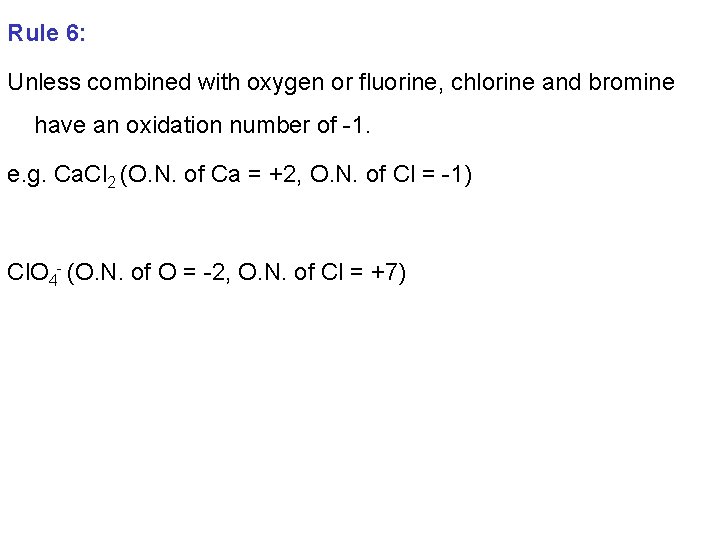
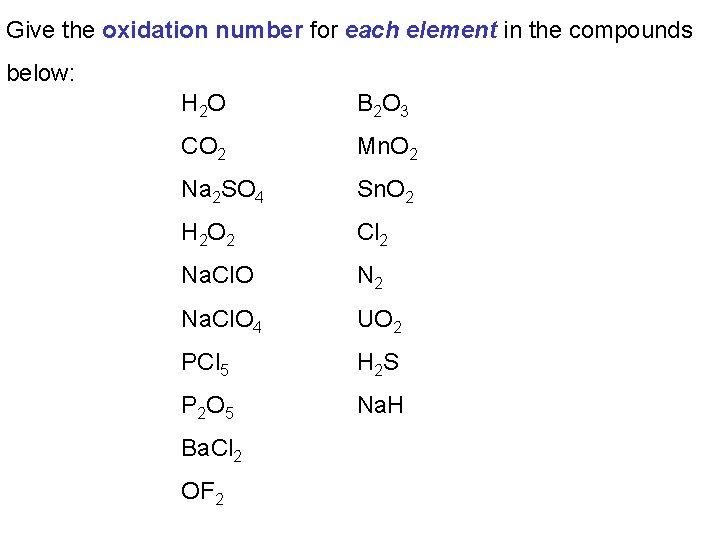
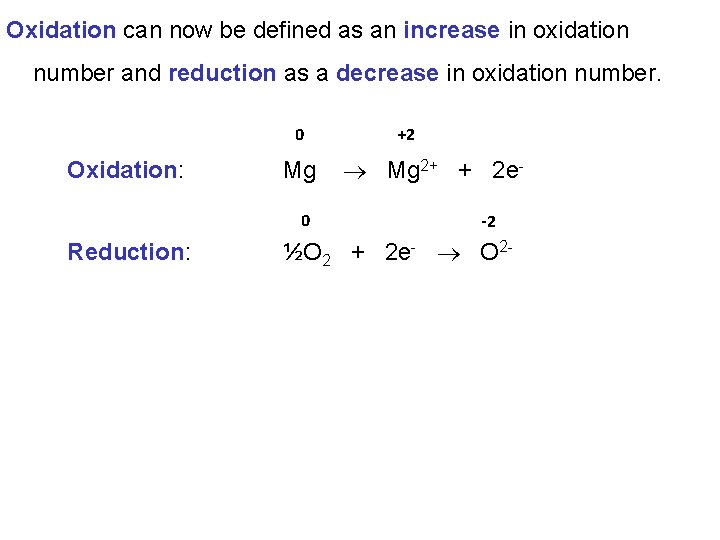
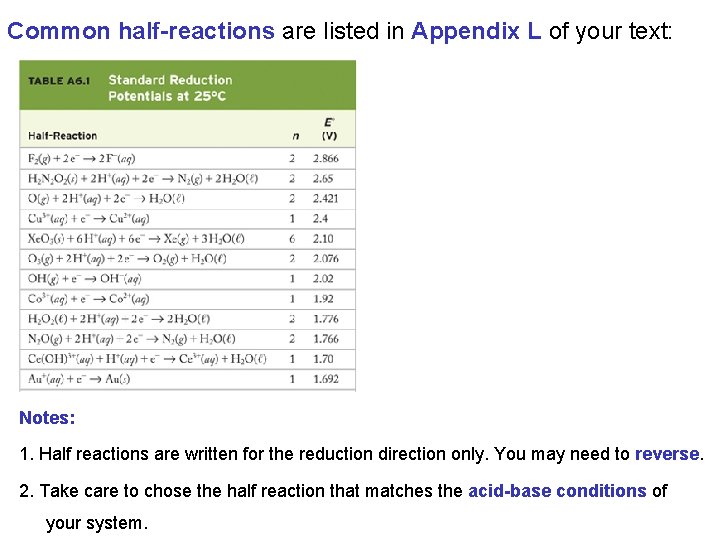
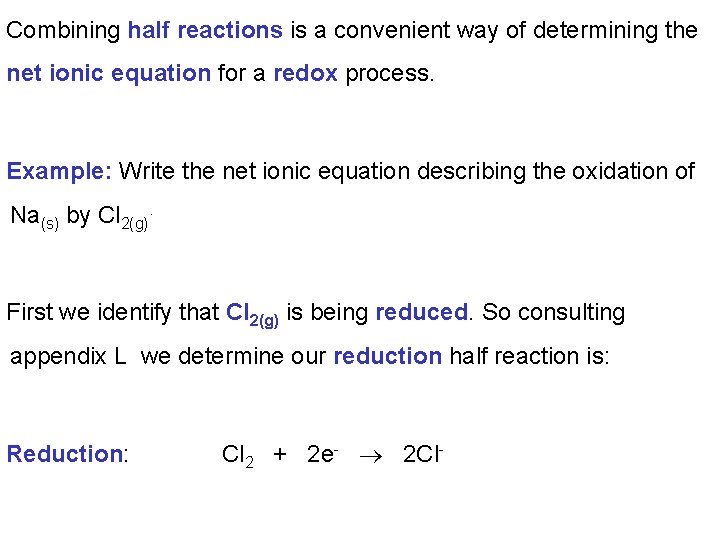
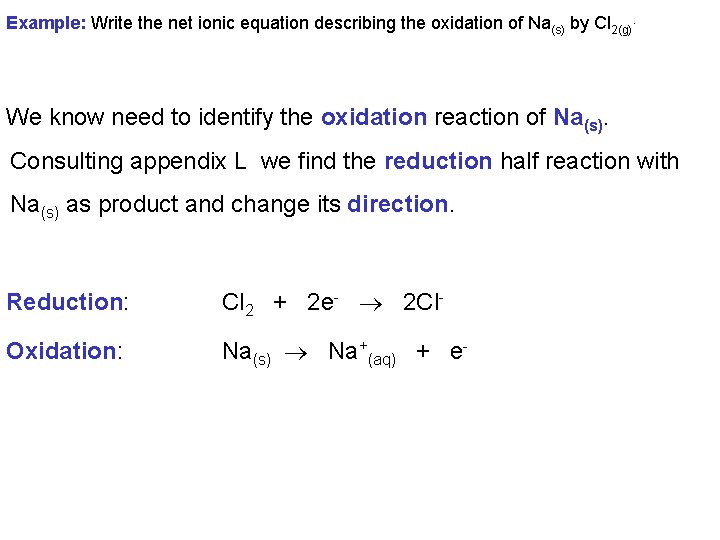
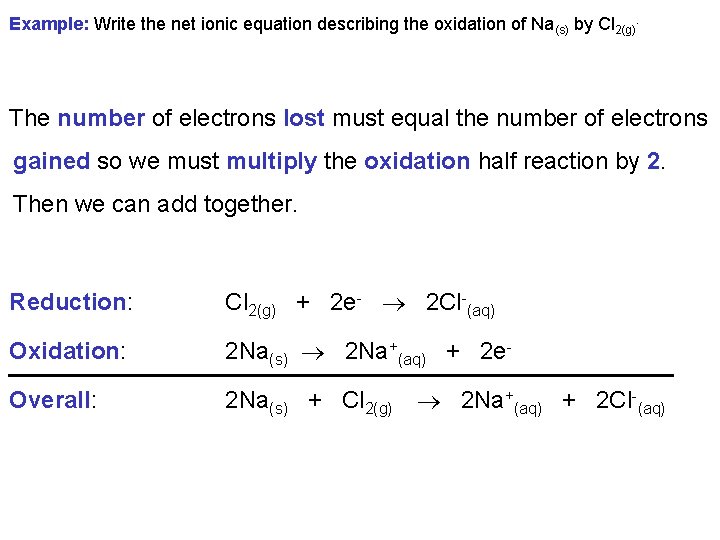
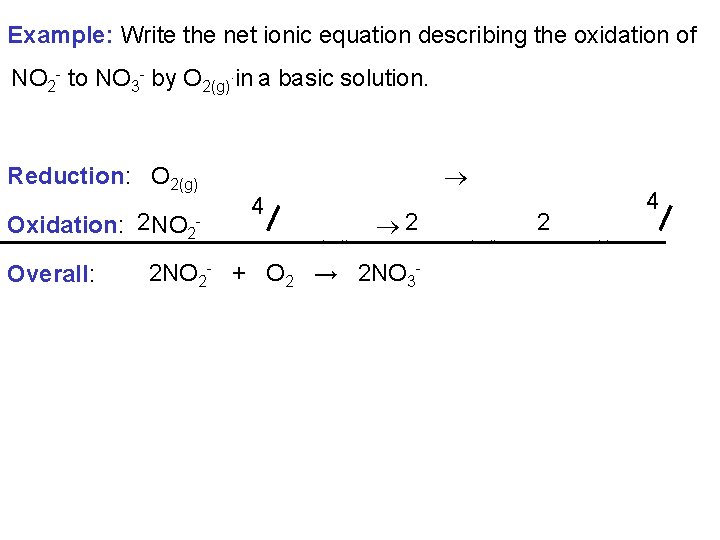
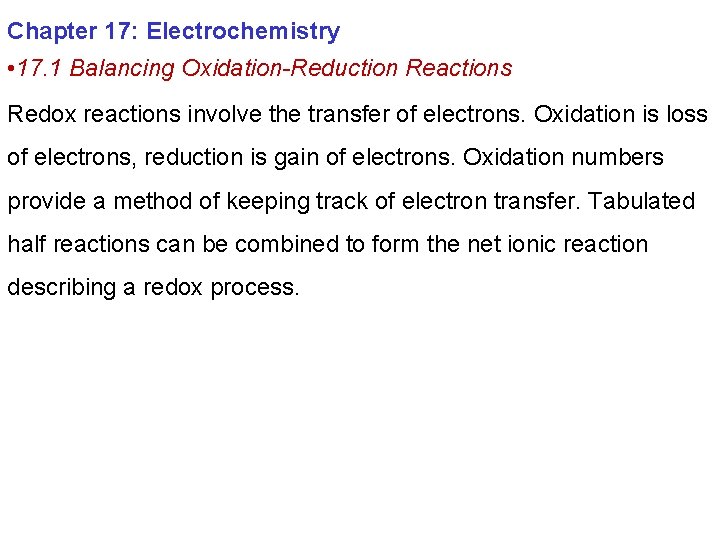
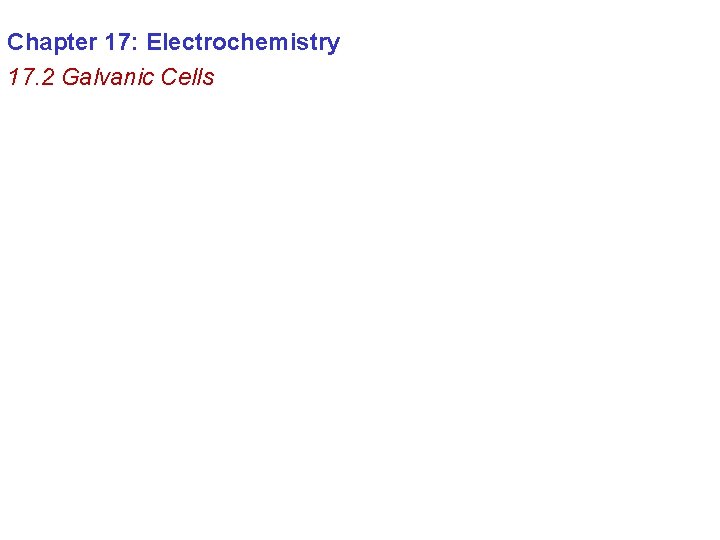
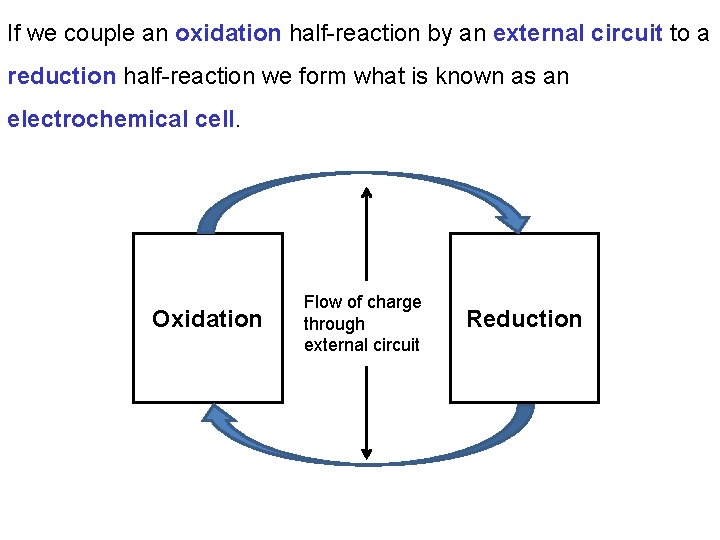
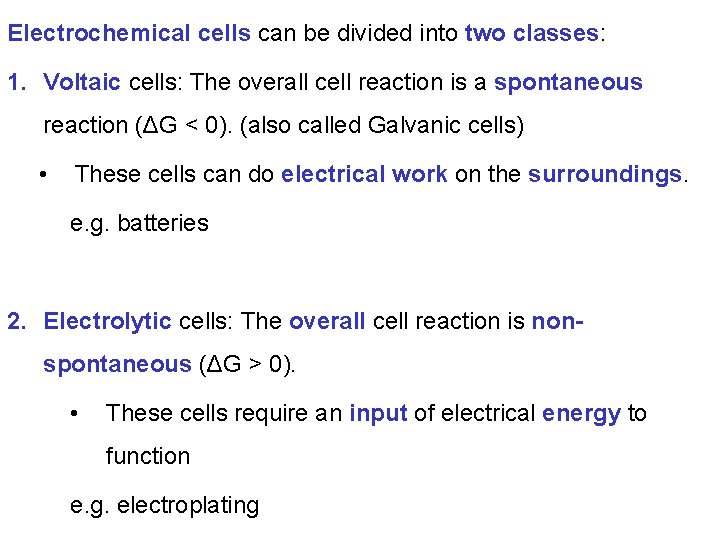
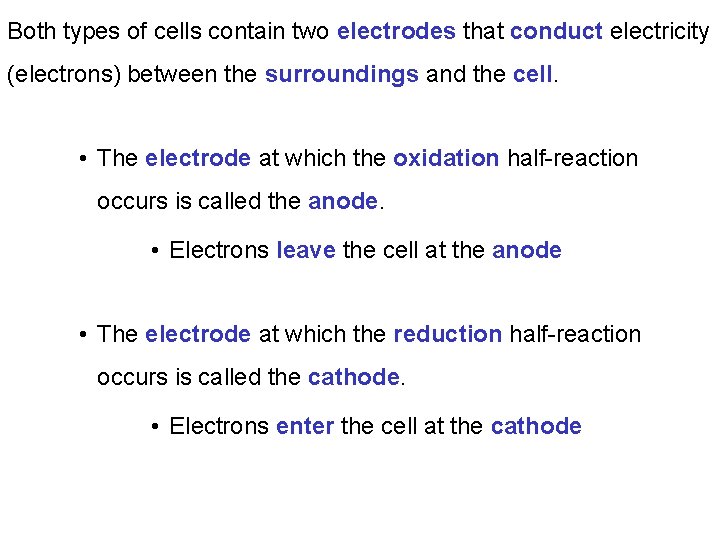
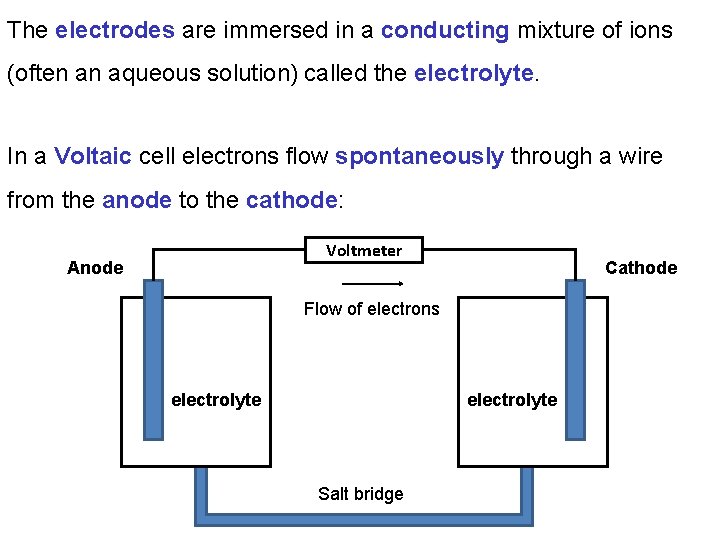
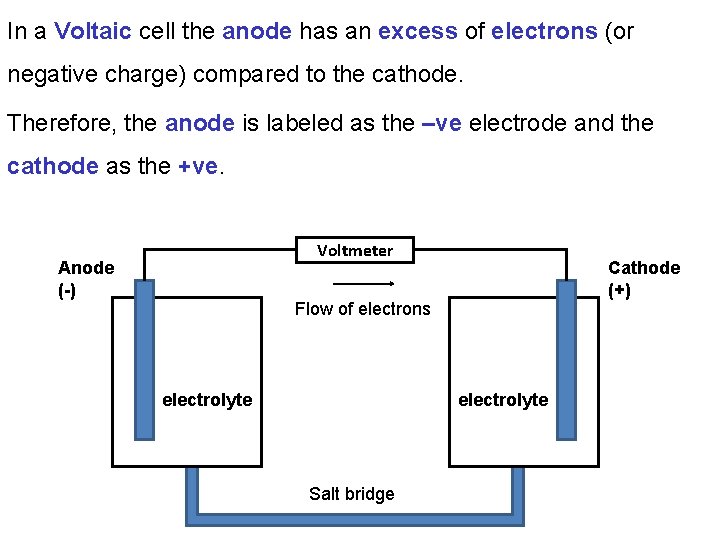
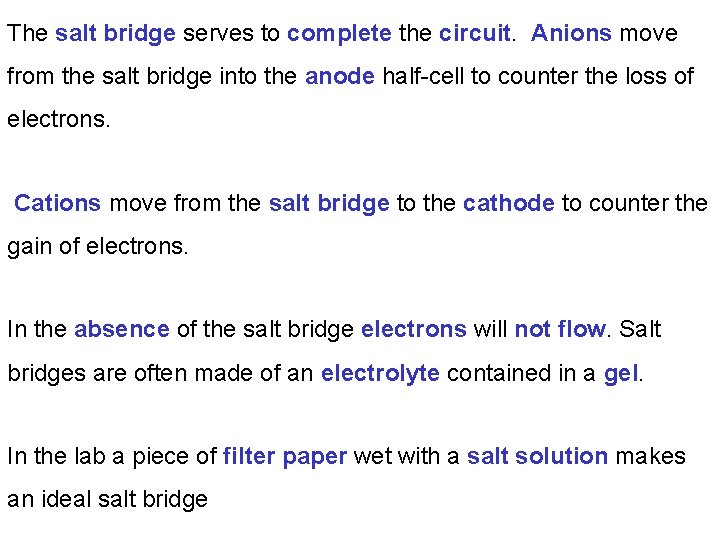
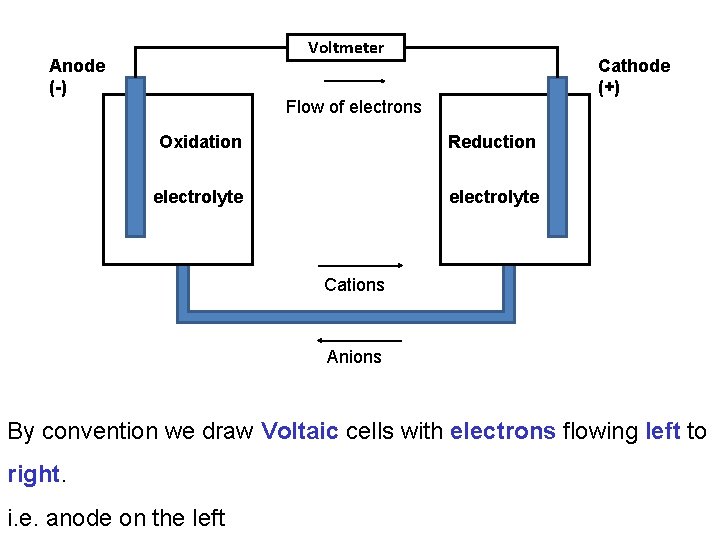
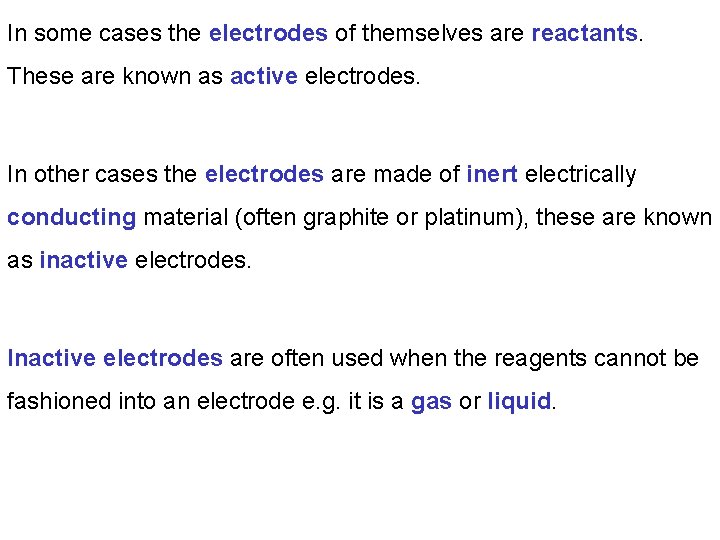
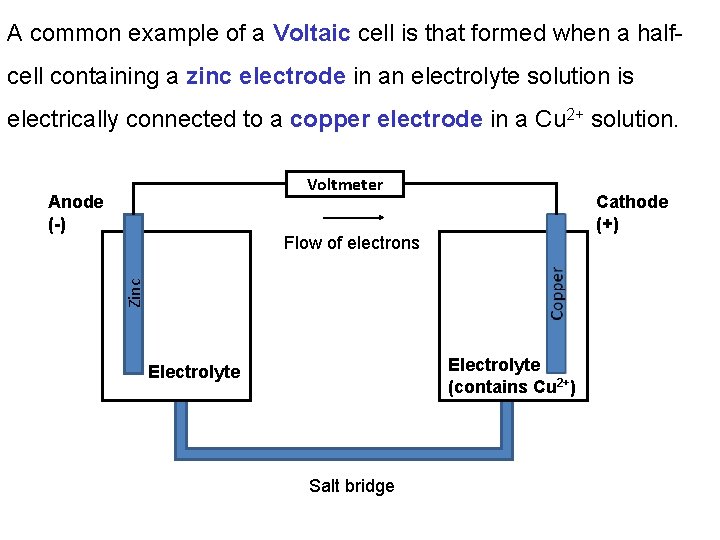
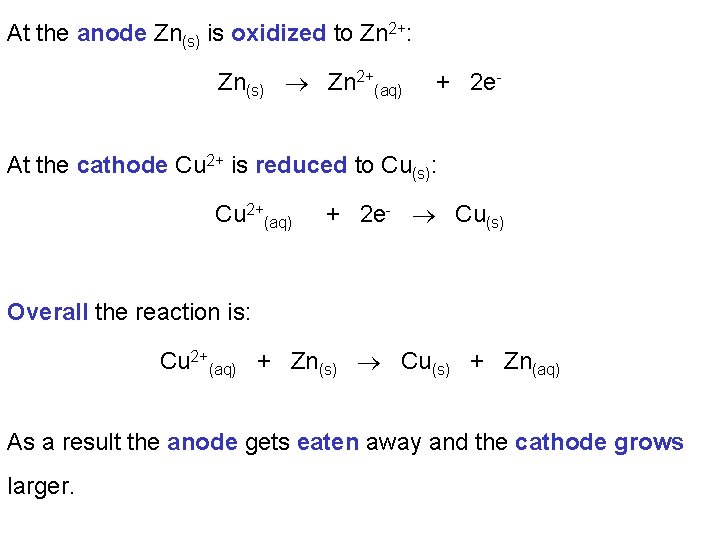
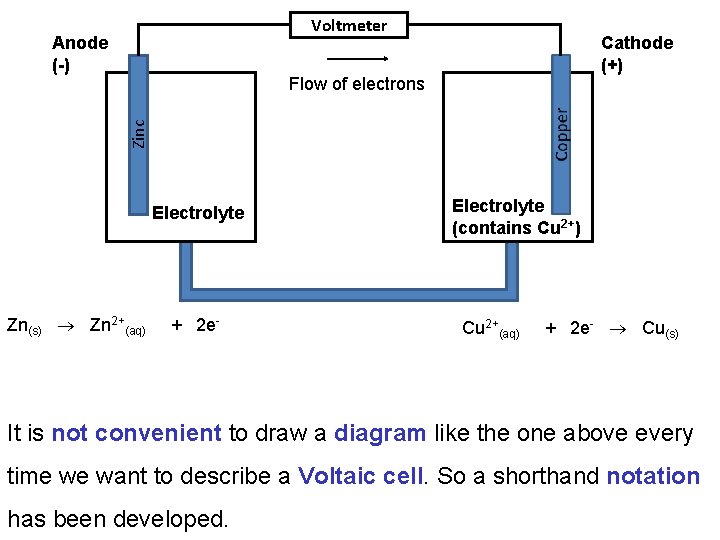
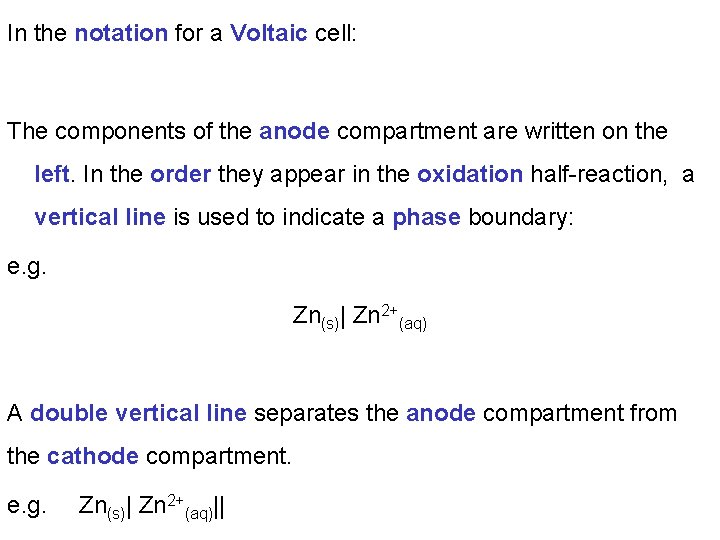
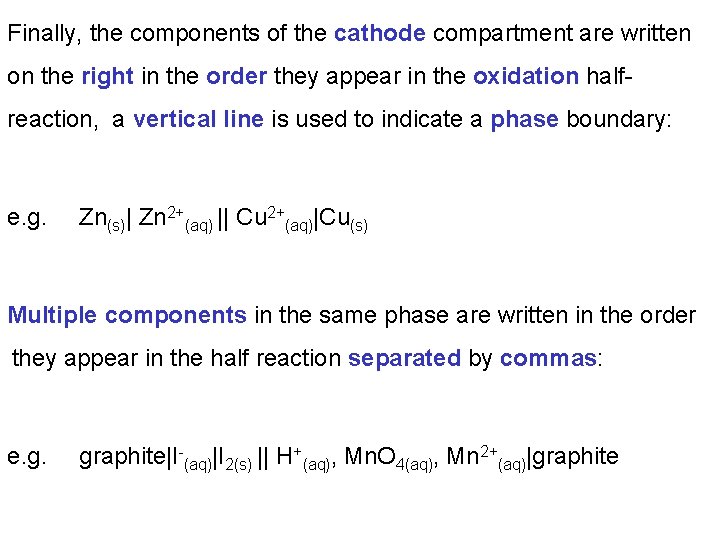
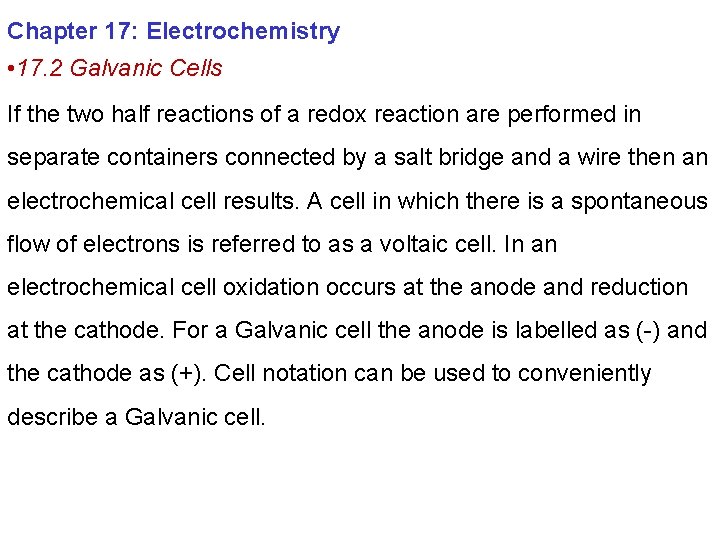
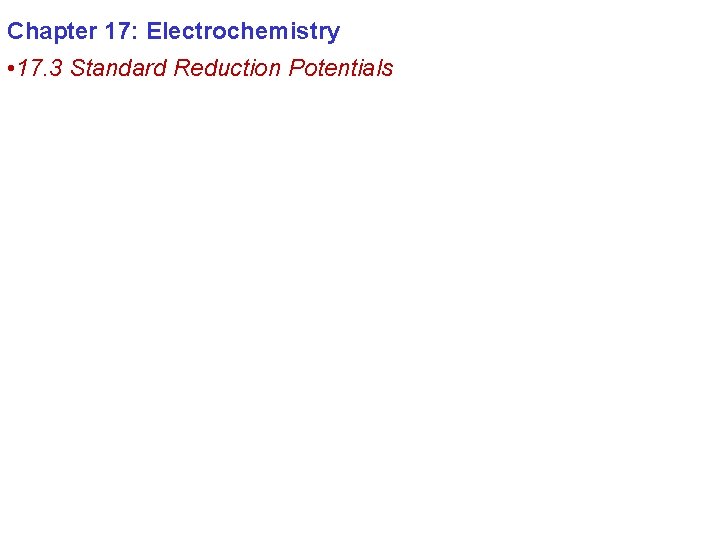
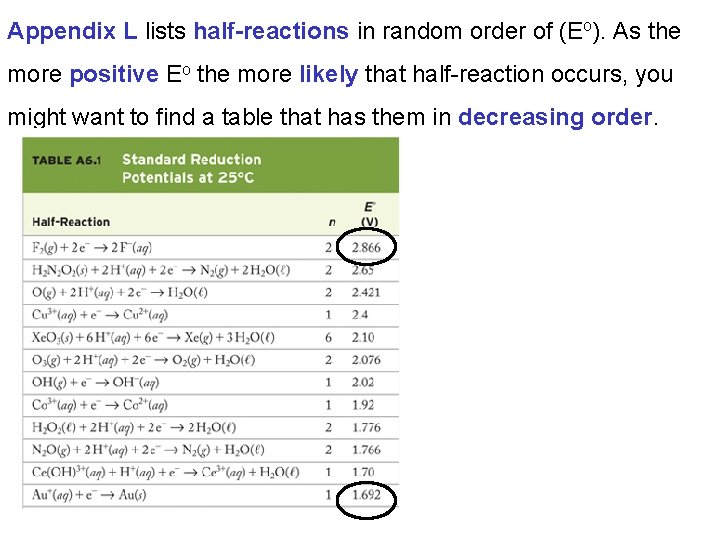
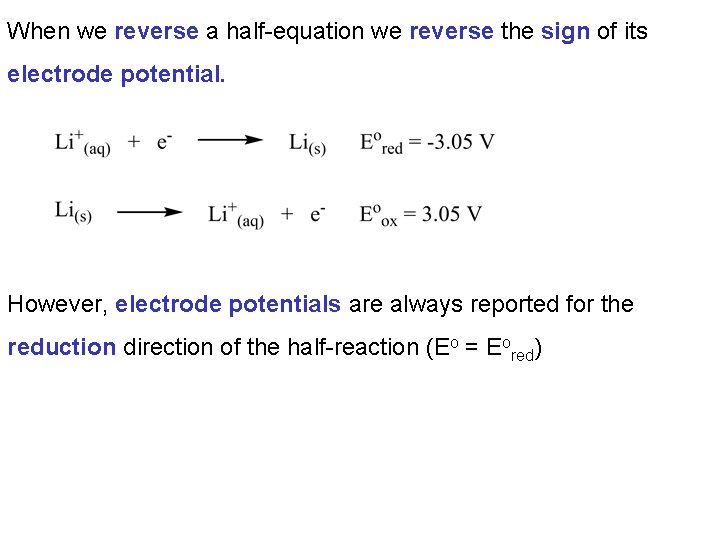
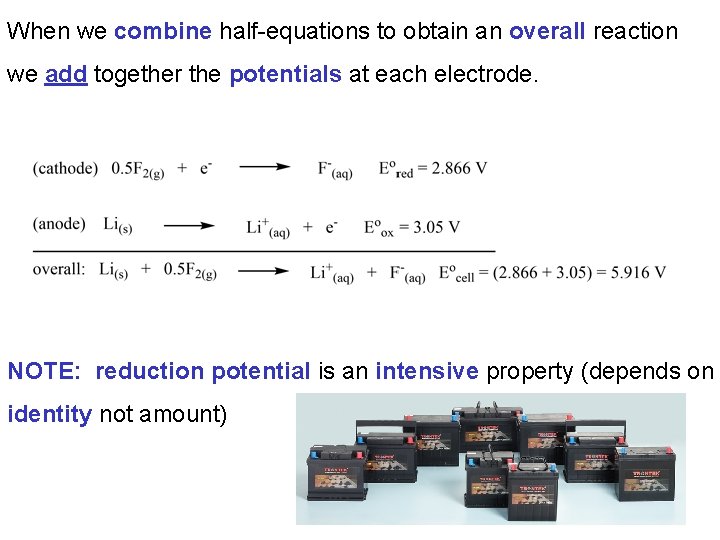
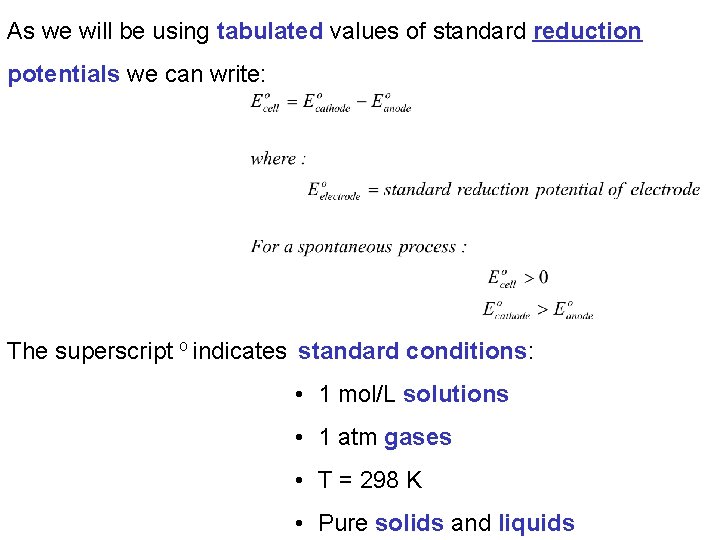
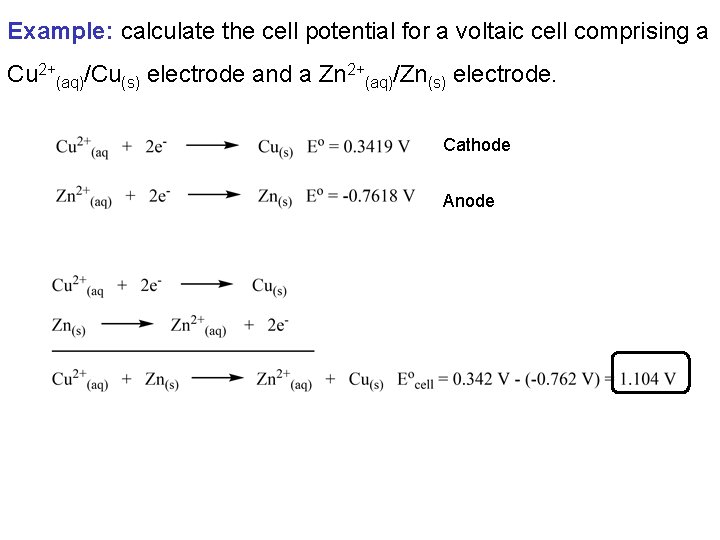
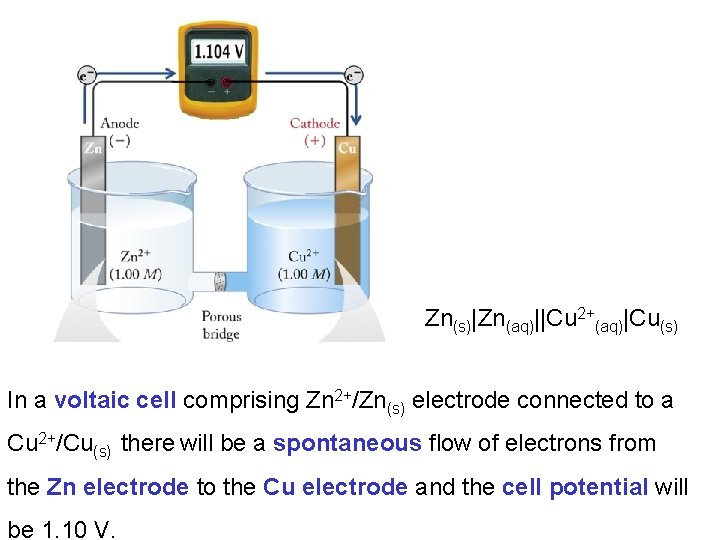
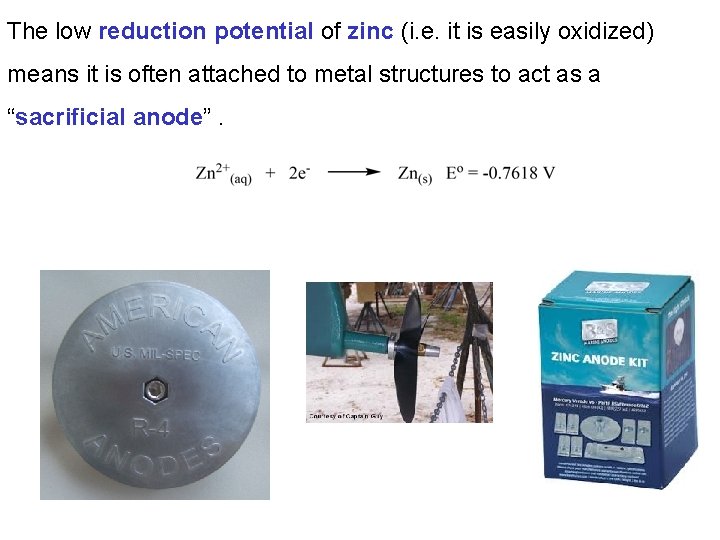
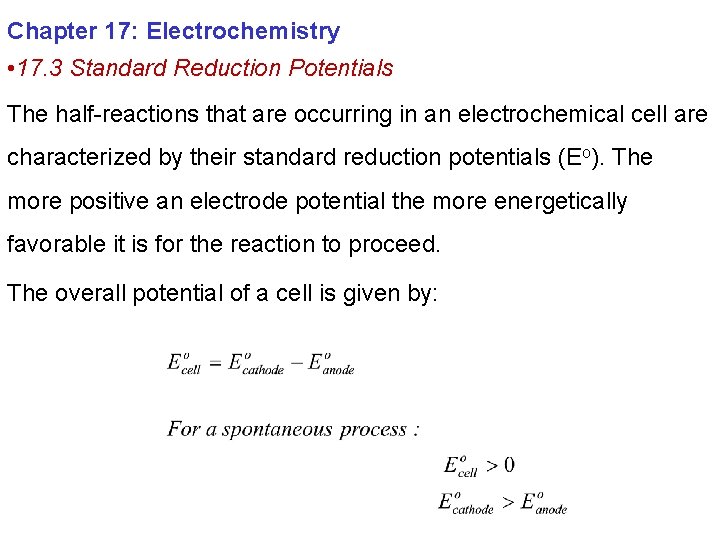
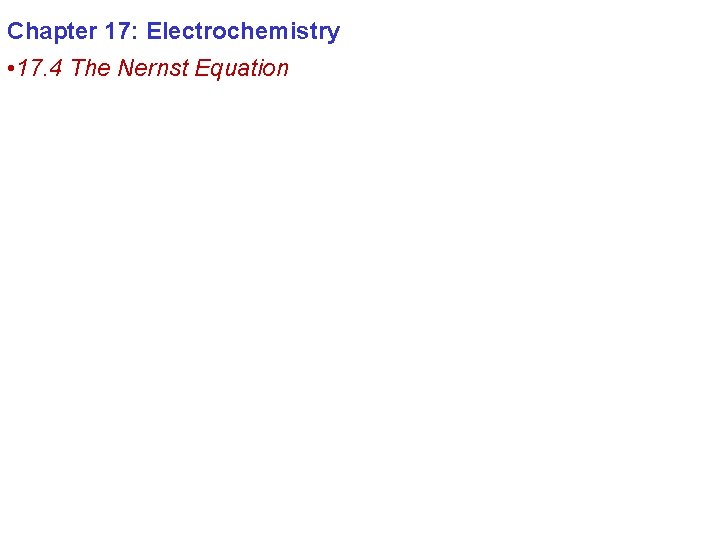
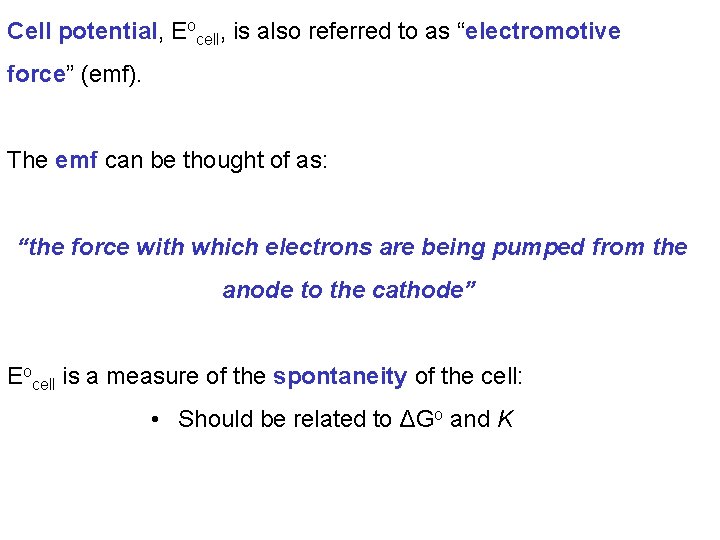
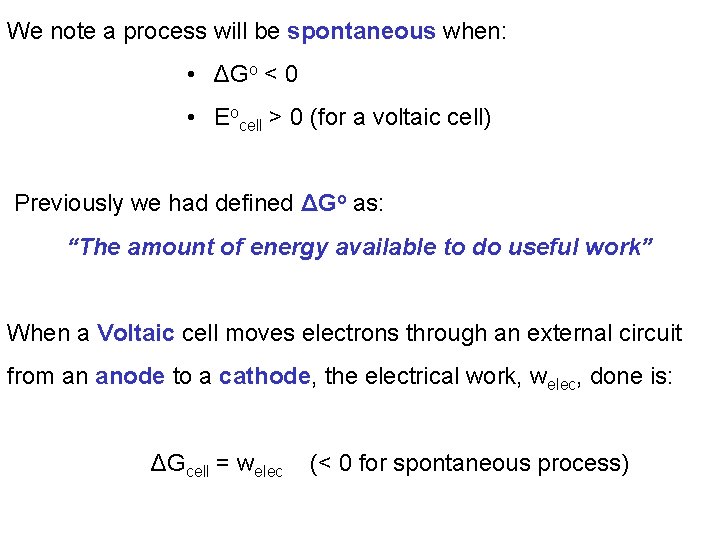
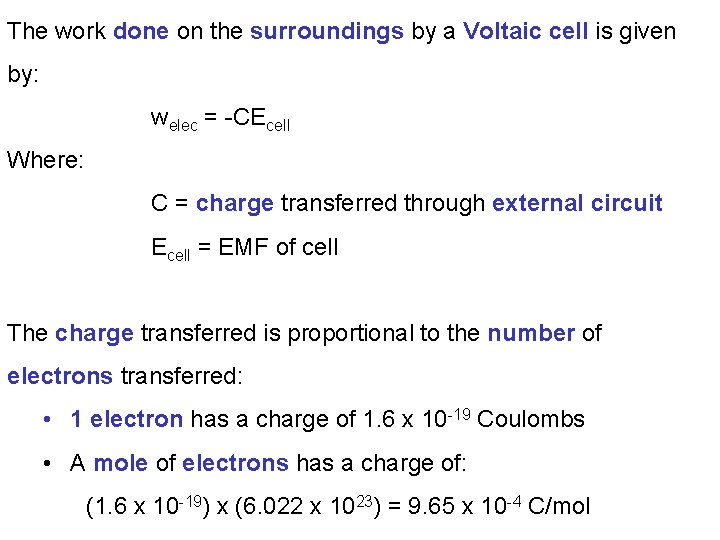
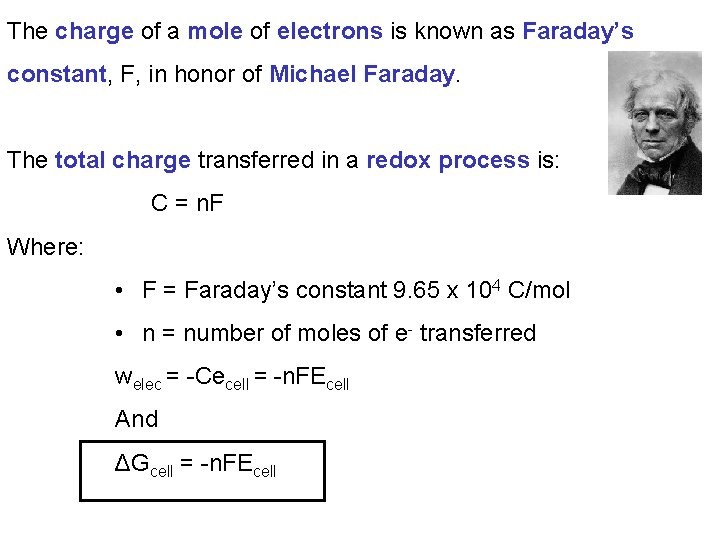
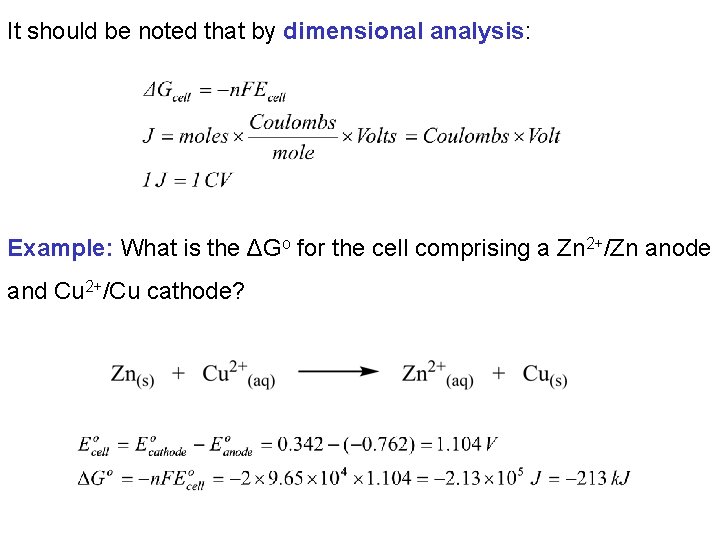
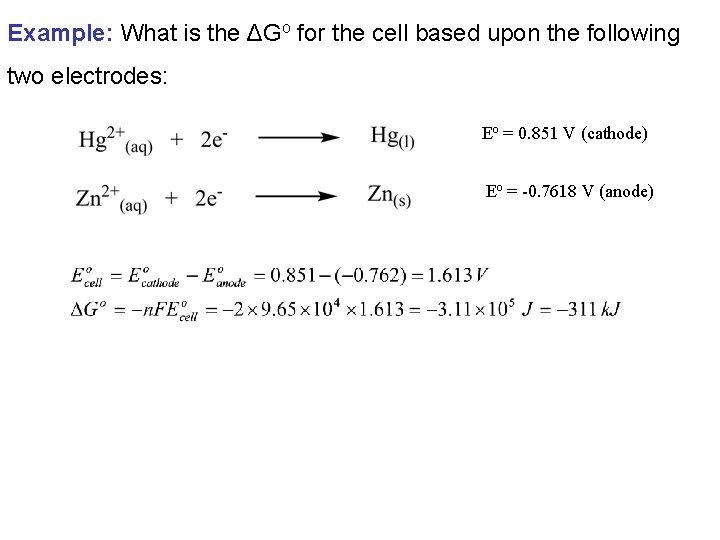
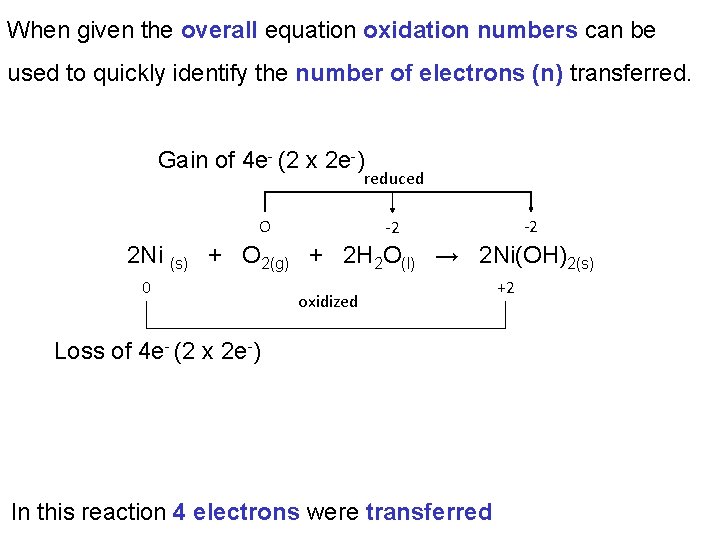
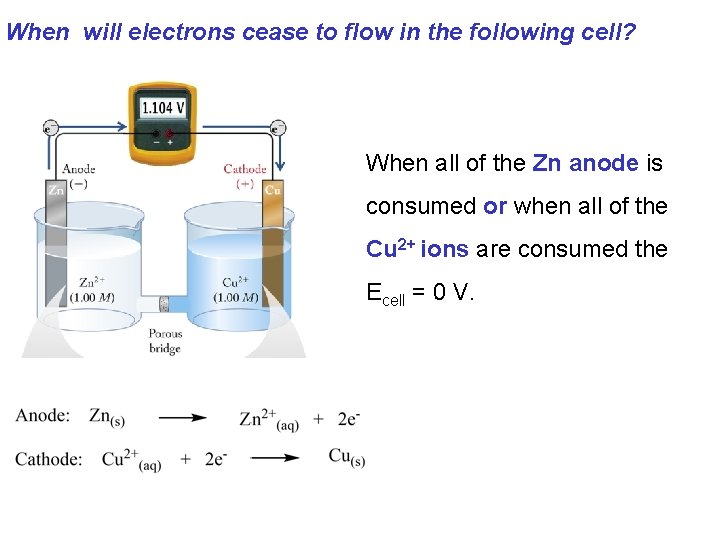
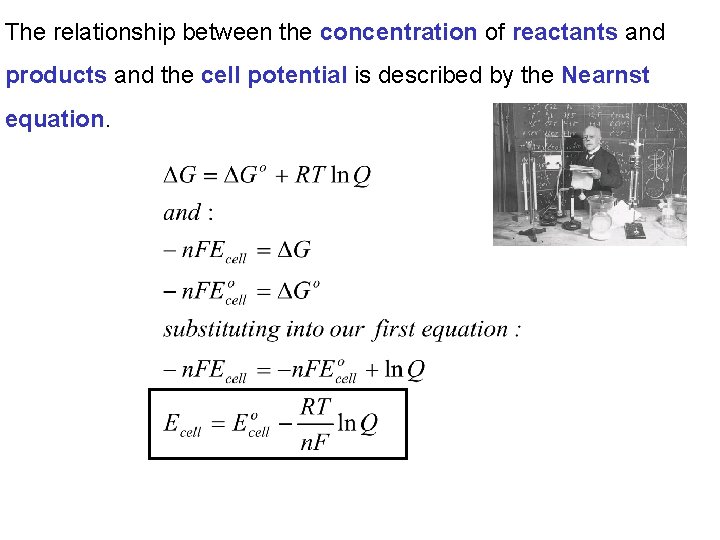
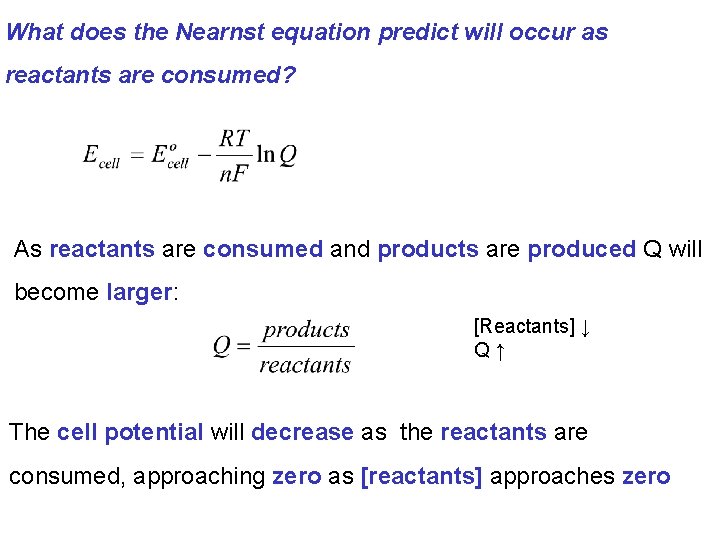
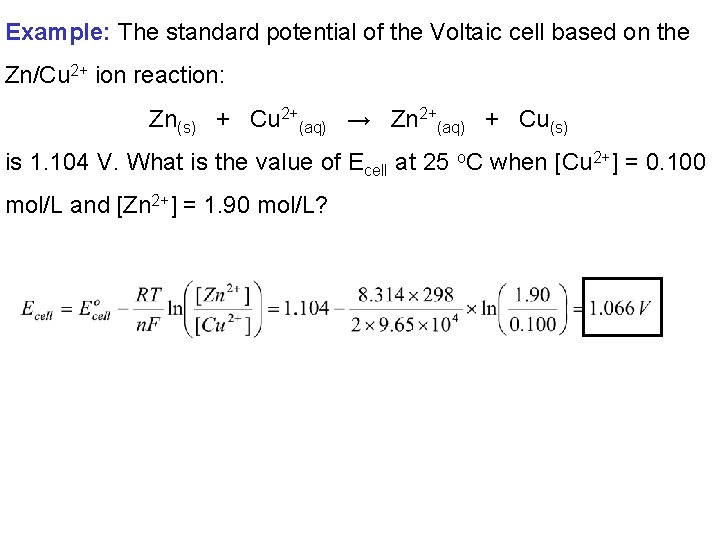
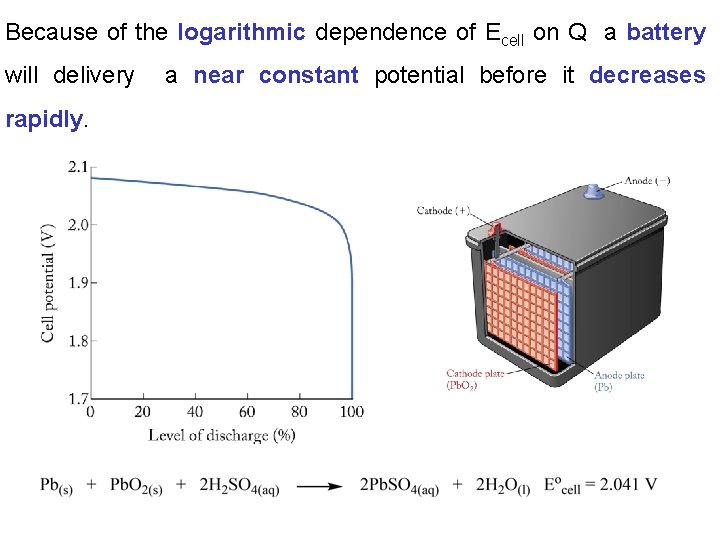
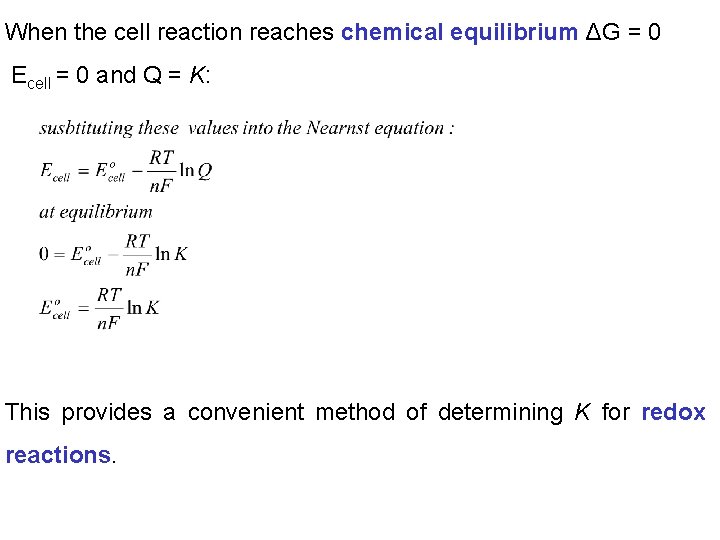
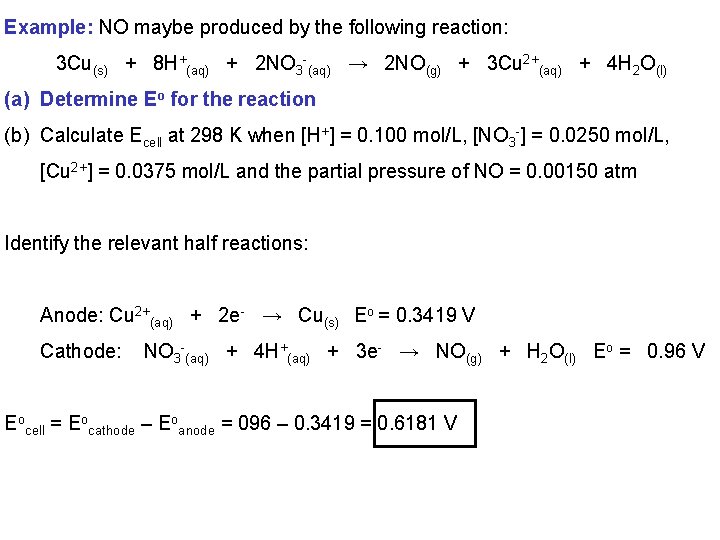
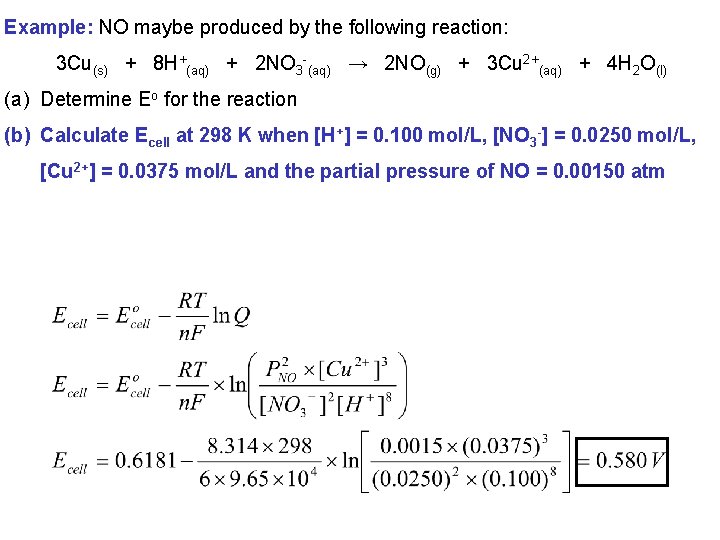
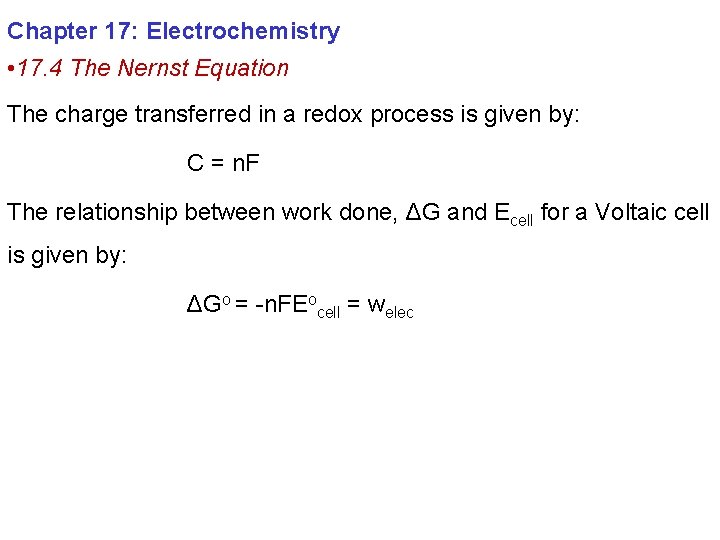
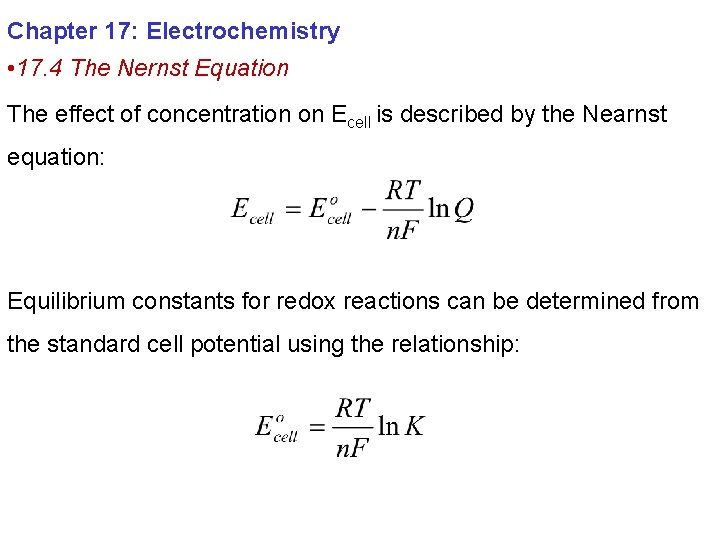
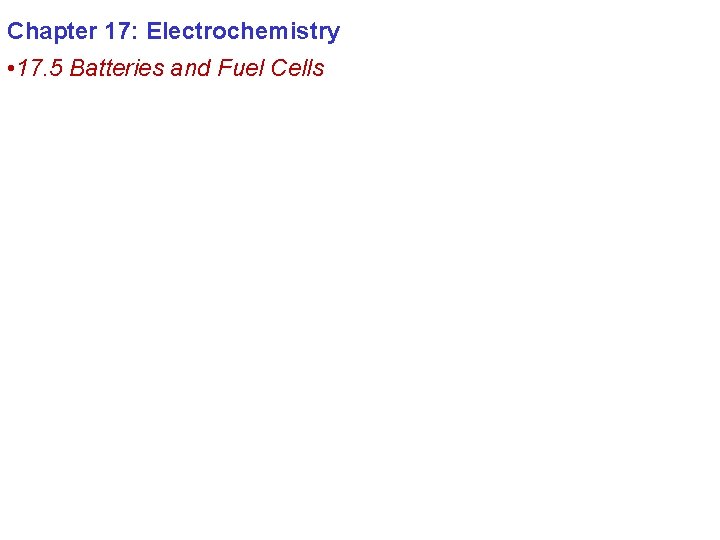
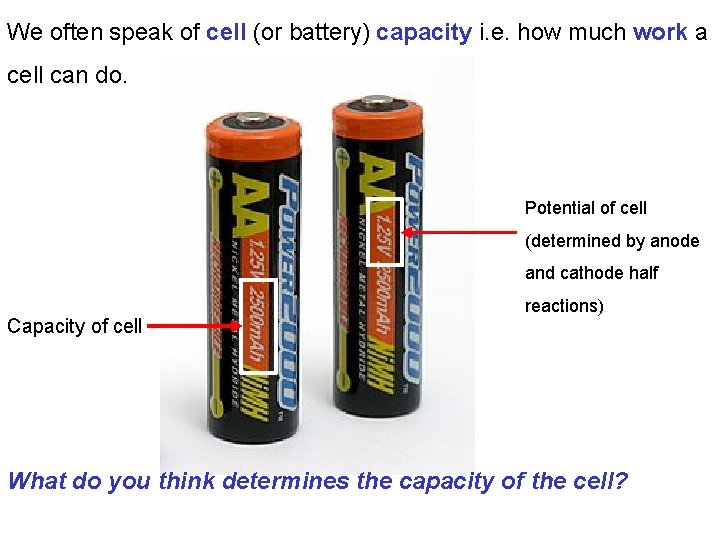
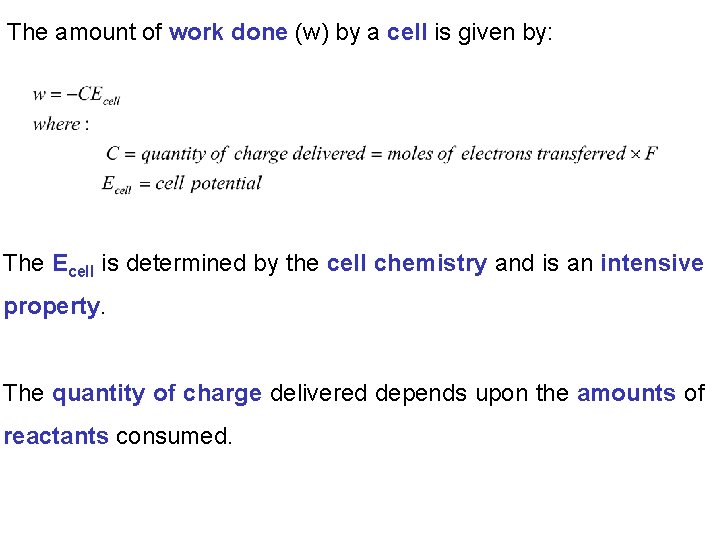
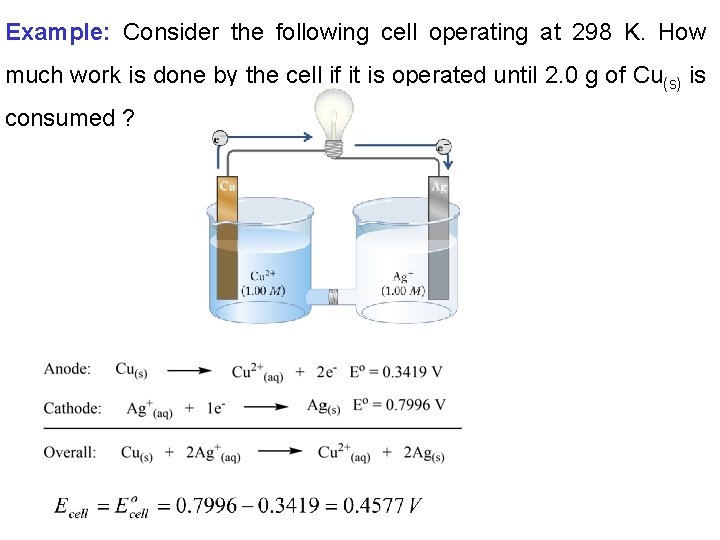
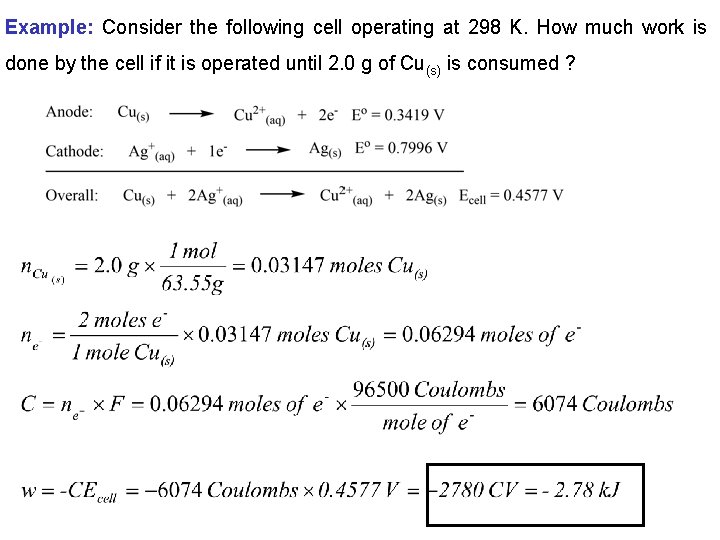
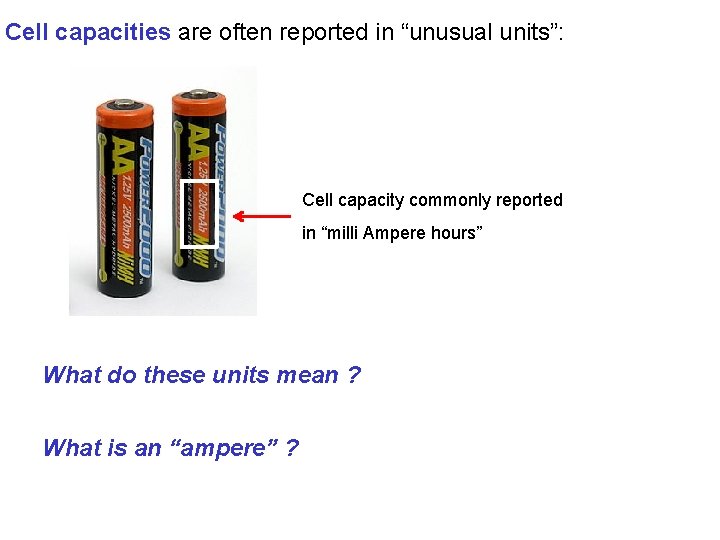
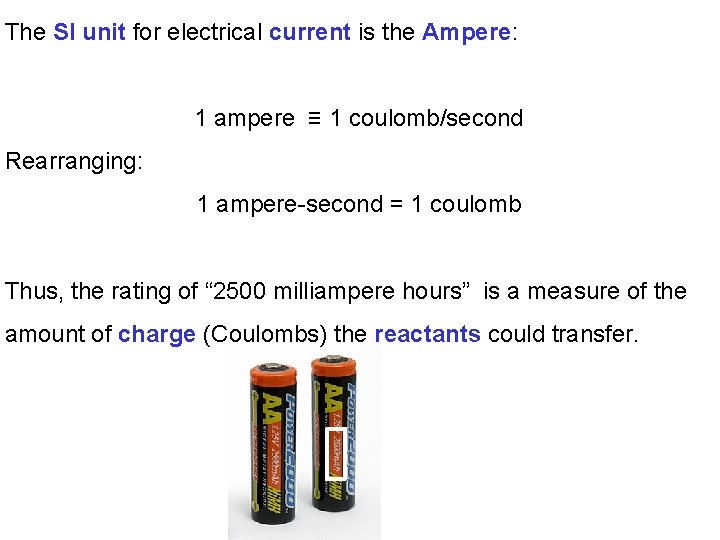
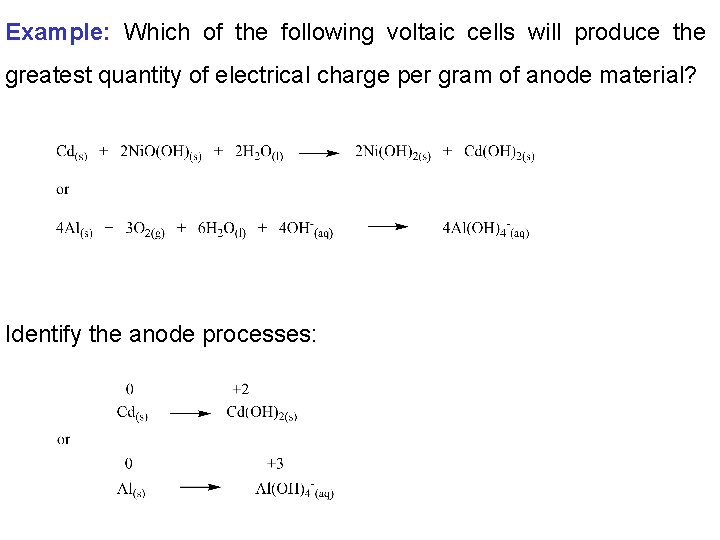
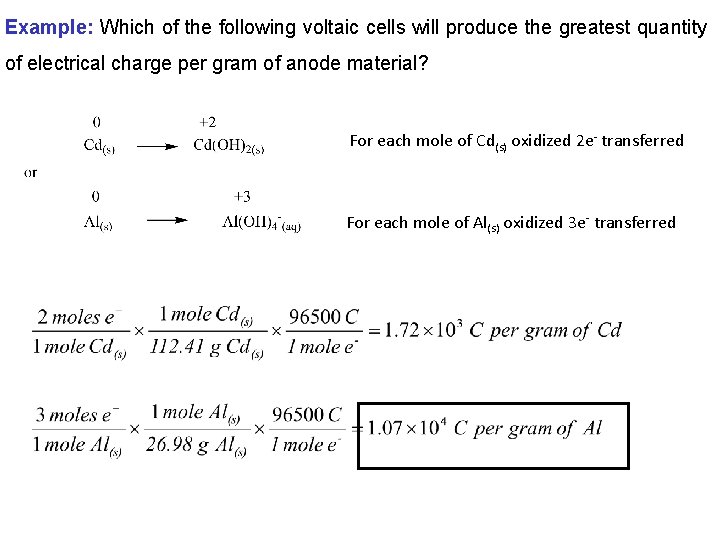
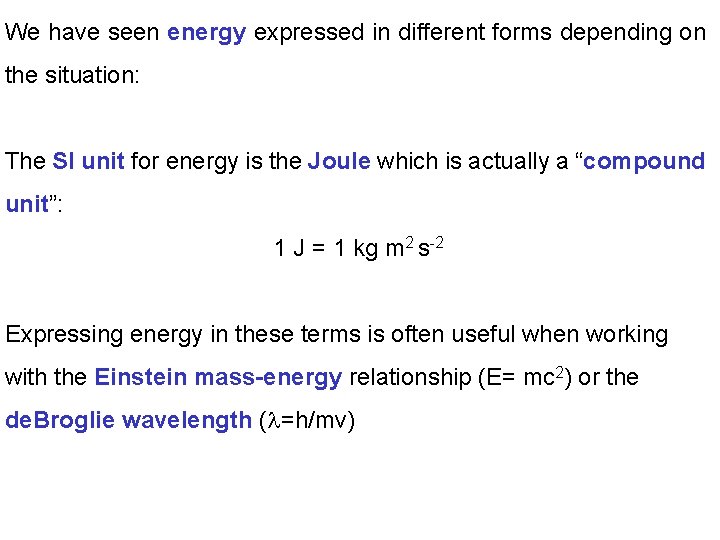
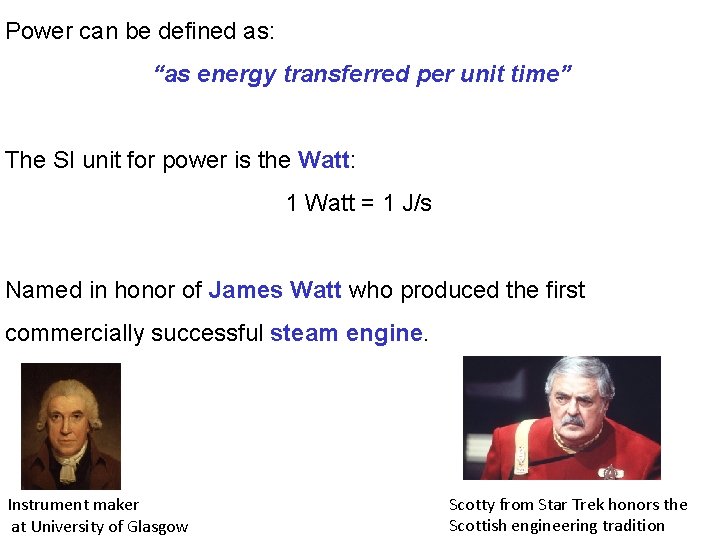
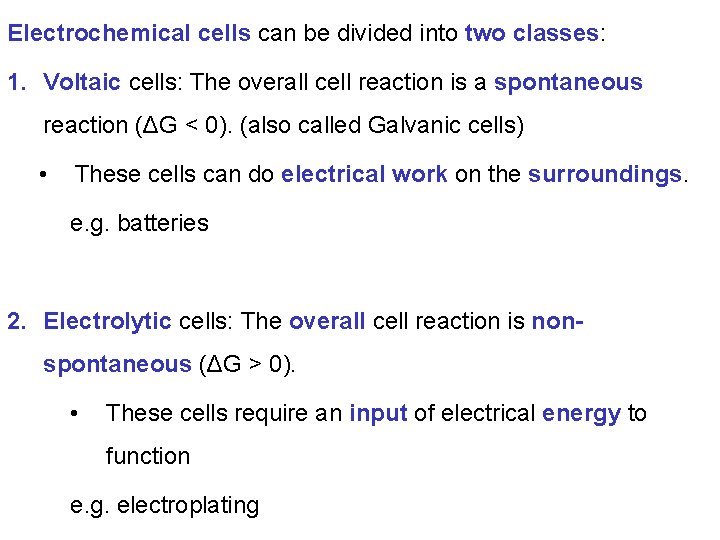
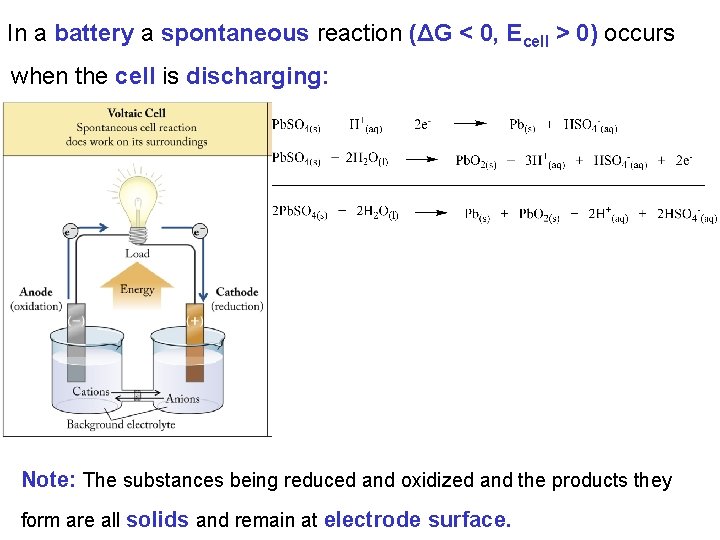
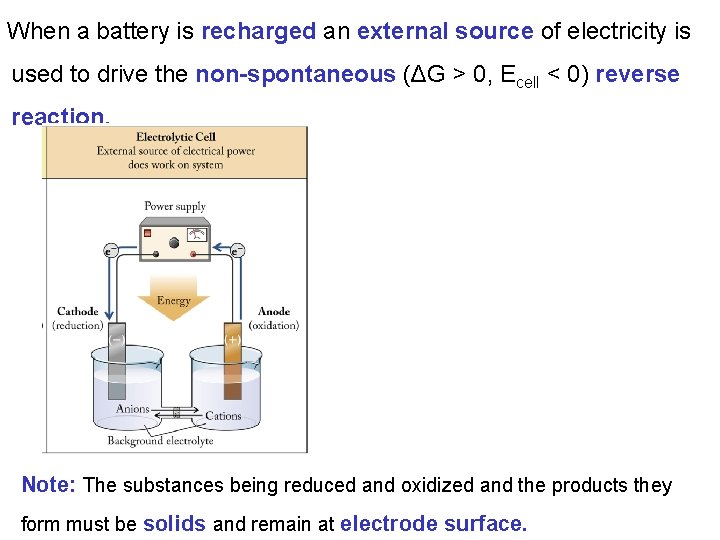
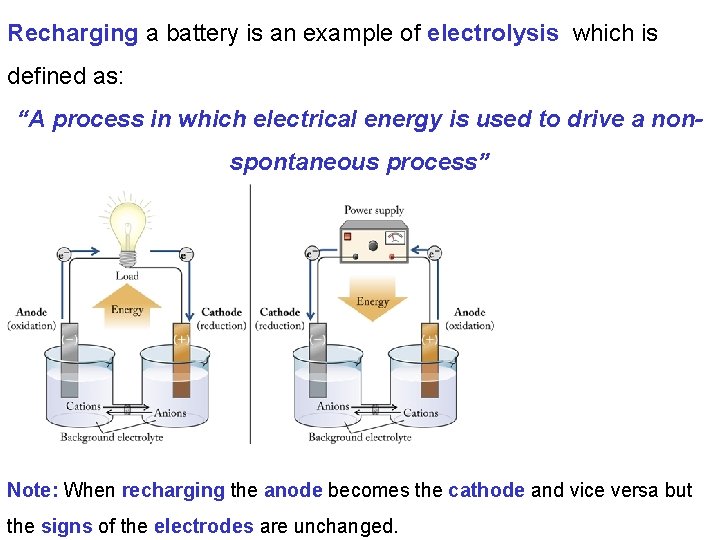
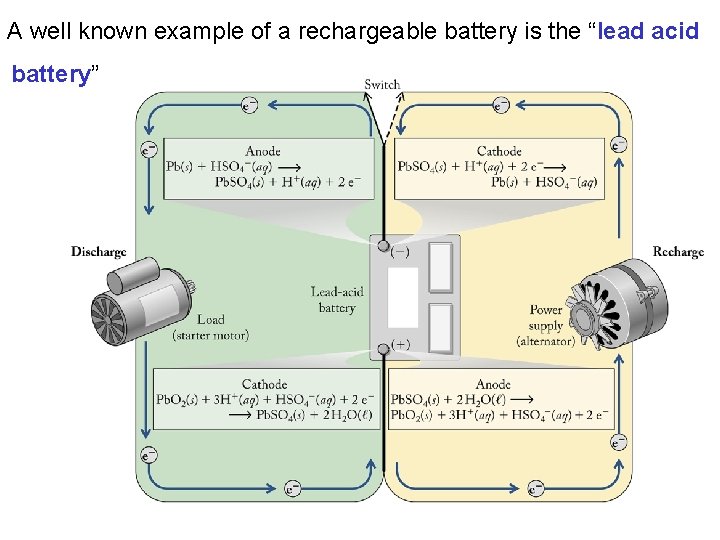
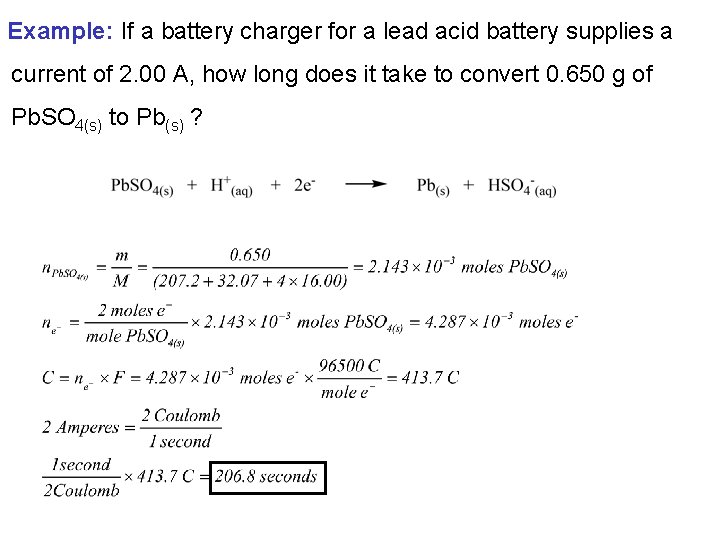
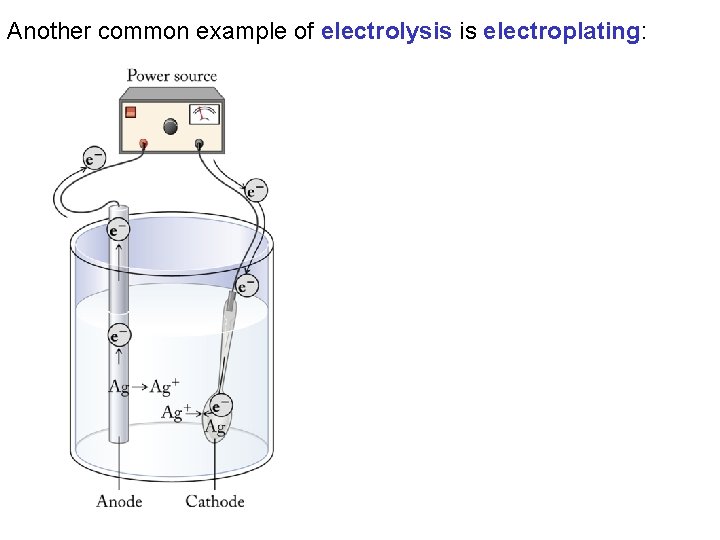
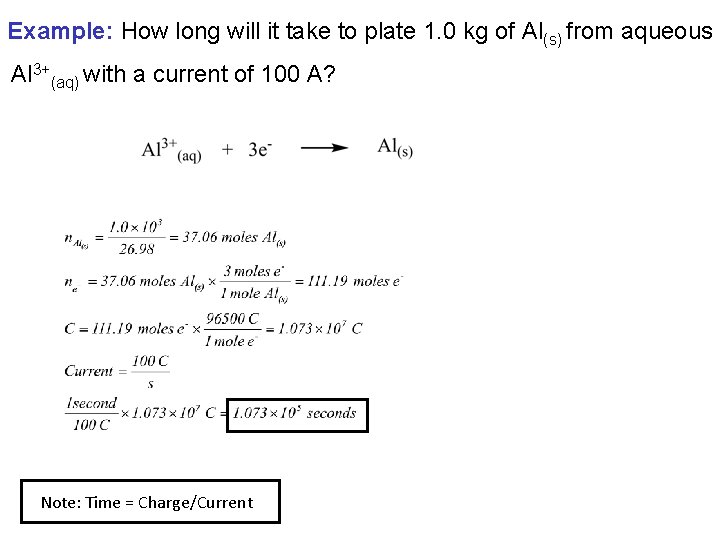
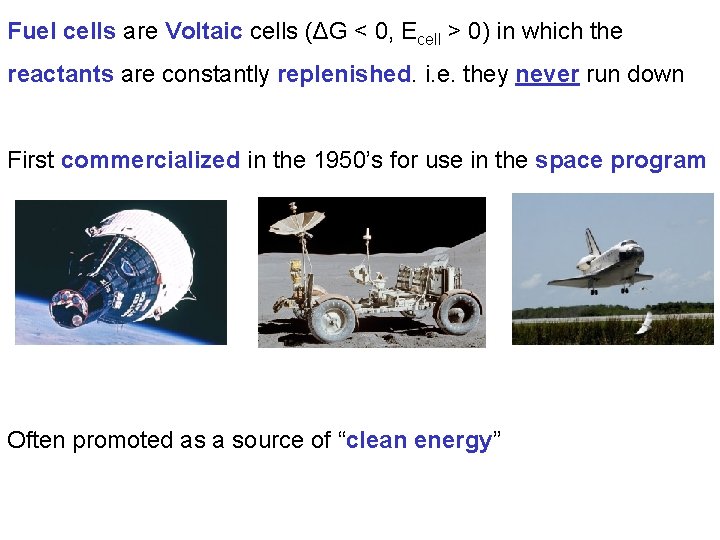
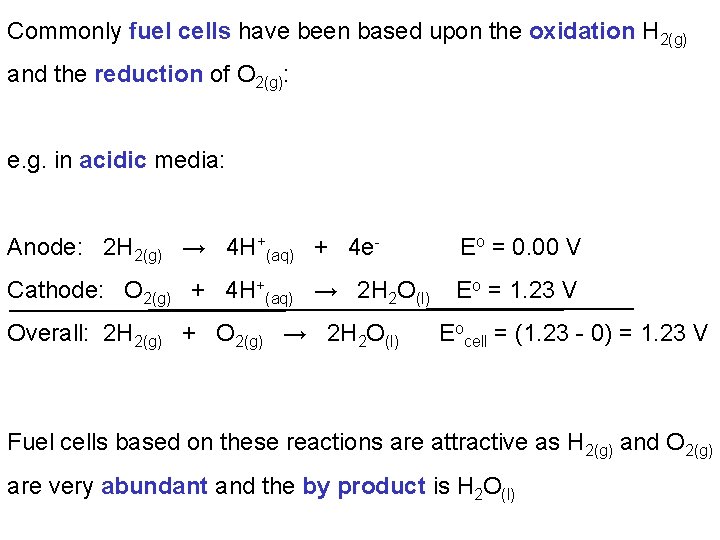
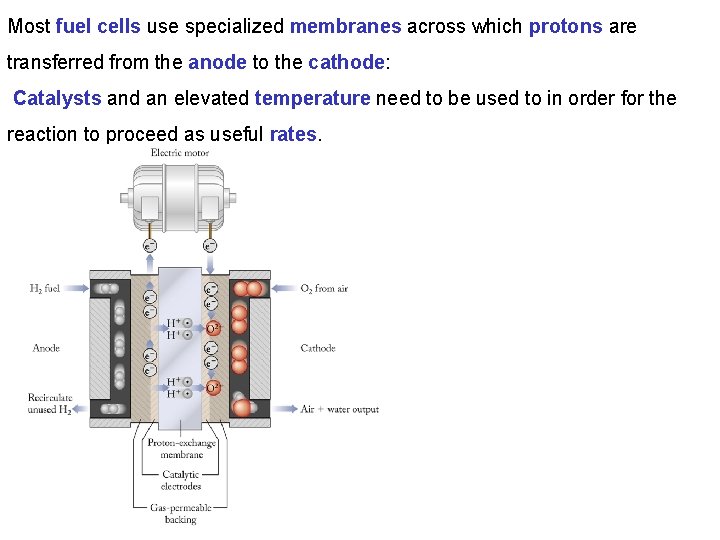
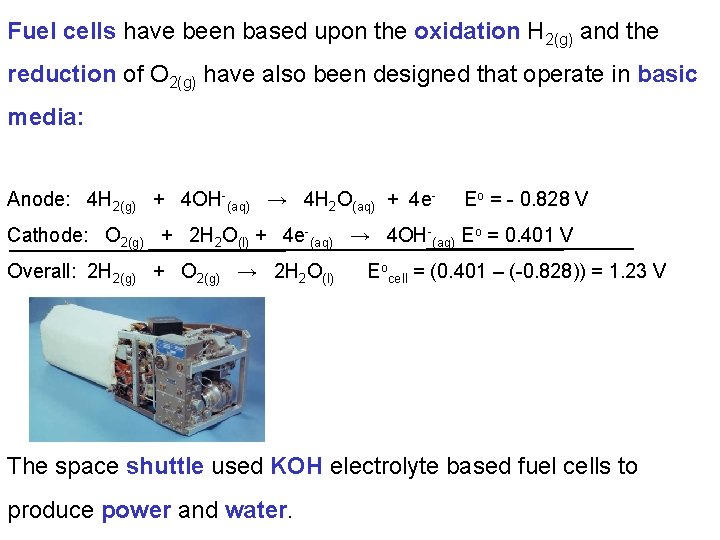
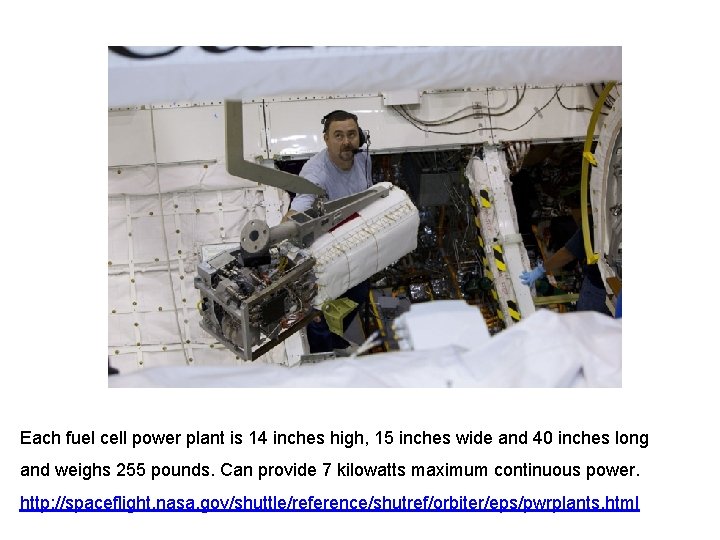
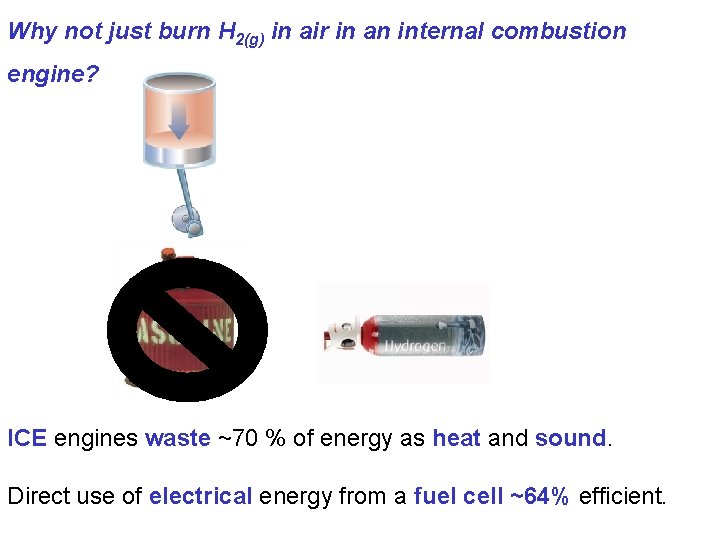
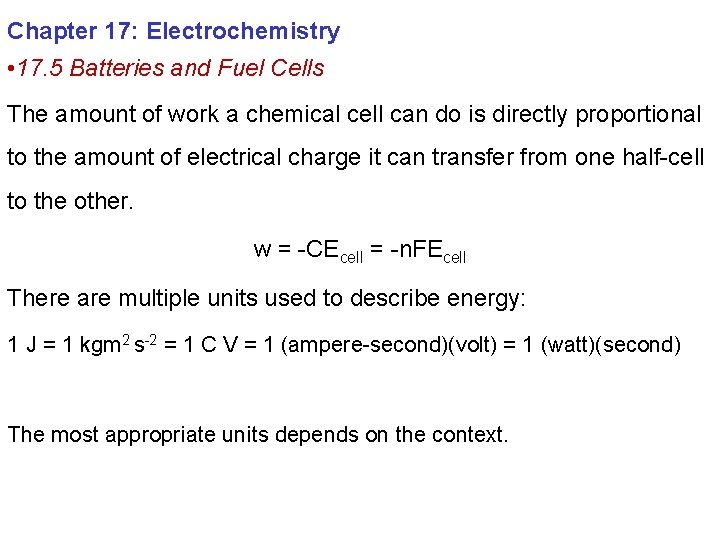
- Slides: 88
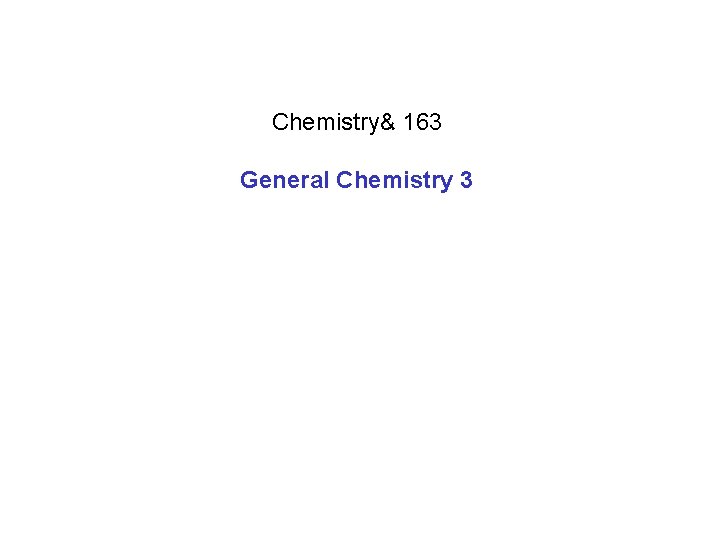
Chemistry& 163 General Chemistry 3
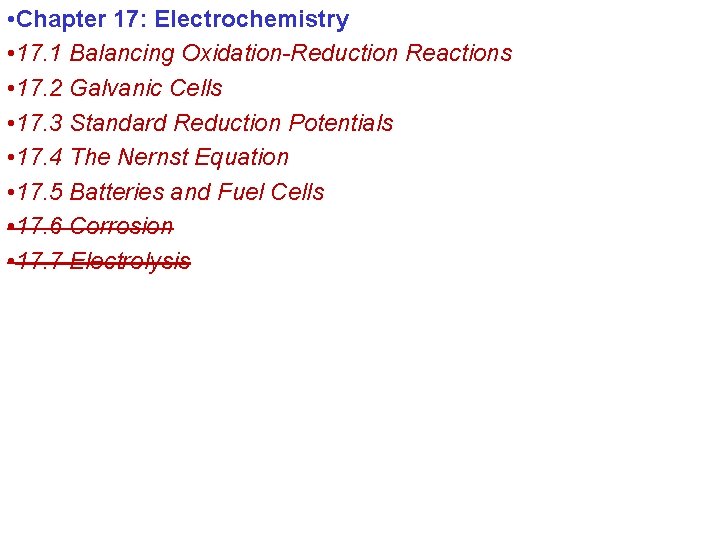
• Chapter 17: Electrochemistry • 17. 1 Balancing Oxidation-Reduction Reactions • 17. 2 Galvanic Cells • 17. 3 Standard Reduction Potentials • 17. 4 The Nernst Equation • 17. 5 Batteries and Fuel Cells • 17. 6 Corrosion • 17. 7 Electrolysis
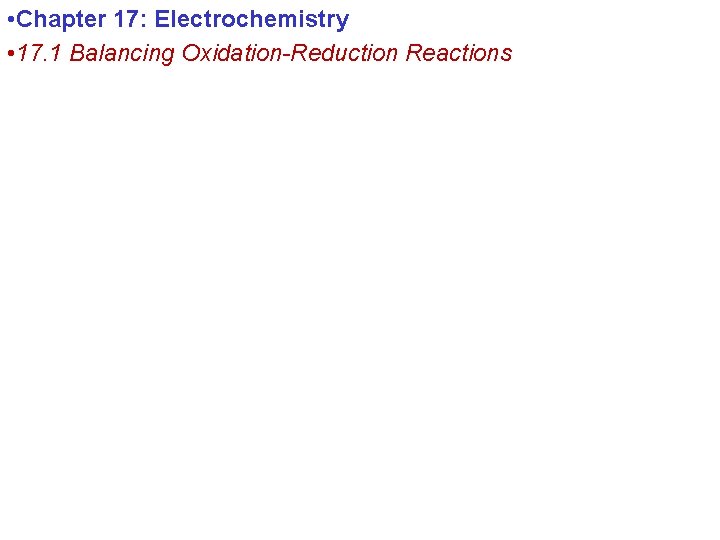
• Chapter 17: Electrochemistry • 17. 1 Balancing Oxidation-Reduction Reactions
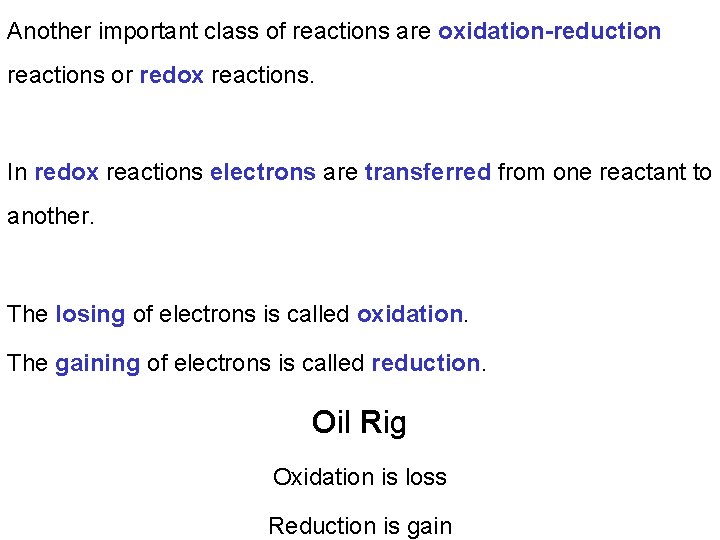
Another important class of reactions are oxidation-reduction reactions or redox reactions. In redox reactions electrons are transferred from one reactant to another. The losing of electrons is called oxidation. The gaining of electrons is called reduction. Oil Rig Oxidation is loss Reduction is gain
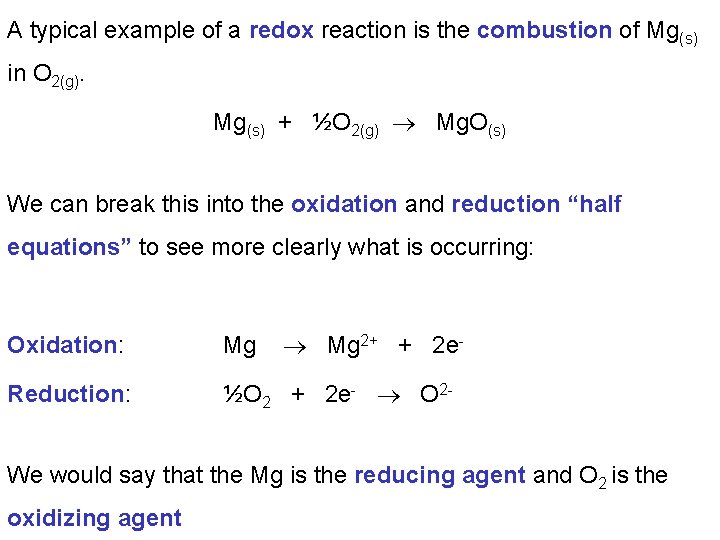
A typical example of a redox reaction is the combustion of Mg(s) in O 2(g). Mg(s) + ½O 2(g) Mg. O(s) We can break this into the oxidation and reduction “half equations” to see more clearly what is occurring: Mg 2+ + 2 e- Oxidation: Mg Reduction: ½O 2 + 2 e- O 2 - We would say that the Mg is the reducing agent and O 2 is the oxidizing agent
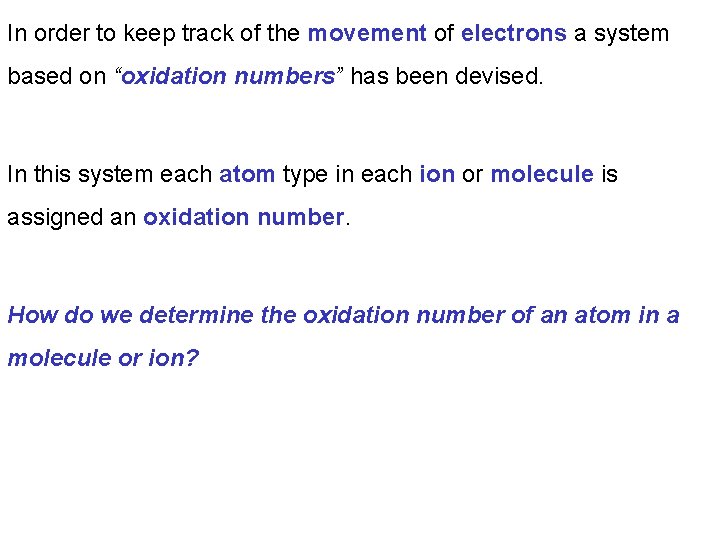
In order to keep track of the movement of electrons a system based on “oxidation numbers” has been devised. In this system each atom type in each ion or molecule is assigned an oxidation number. How do we determine the oxidation number of an atom in a molecule or ion?
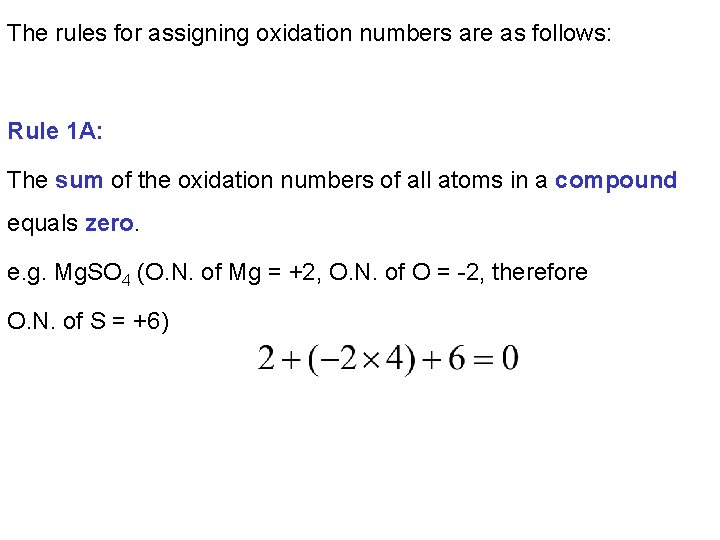
The rules for assigning oxidation numbers are as follows: Rule 1 A: The sum of the oxidation numbers of all atoms in a compound equals zero. e. g. Mg. SO 4 (O. N. of Mg = +2, O. N. of O = -2, therefore O. N. of S = +6)
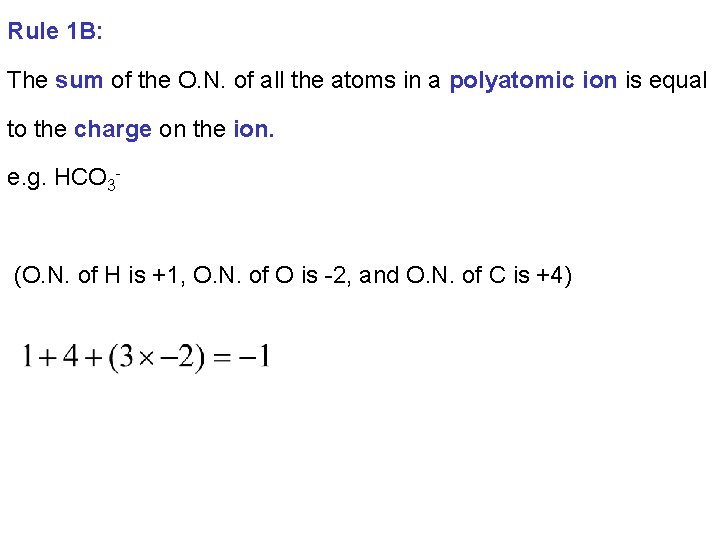
Rule 1 B: The sum of the O. N. of all the atoms in a polyatomic ion is equal to the charge on the ion. e. g. HCO 3 - (O. N. of H is +1, O. N. of O is -2, and O. N. of C is +4)
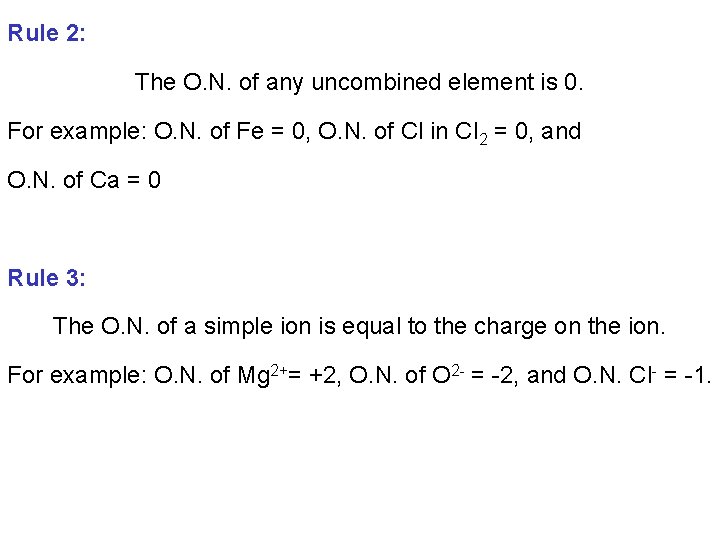
Rule 2: The O. N. of any uncombined element is 0. For example: O. N. of Fe = 0, O. N. of Cl in Cl 2 = 0, and O. N. of Ca = 0 Rule 3: The O. N. of a simple ion is equal to the charge on the ion. For example: O. N. of Mg 2+= +2, O. N. of O 2 - = -2, and O. N. Cl- = -1.
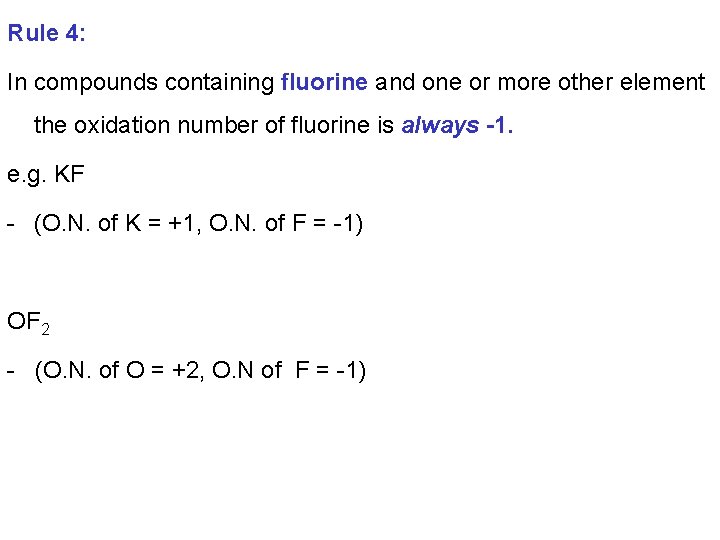
Rule 4: In compounds containing fluorine and one or more other element the oxidation number of fluorine is always -1. e. g. KF - (O. N. of K = +1, O. N. of F = -1) OF 2 - (O. N. of O = +2, O. N of F = -1)
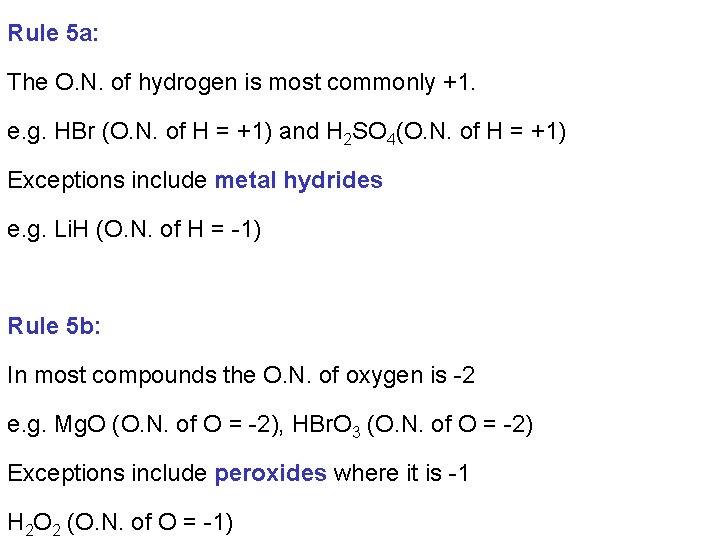
Rule 5 a: The O. N. of hydrogen is most commonly +1. e. g. HBr (O. N. of H = +1) and H 2 SO 4(O. N. of H = +1) Exceptions include metal hydrides e. g. Li. H (O. N. of H = -1) Rule 5 b: In most compounds the O. N. of oxygen is -2 e. g. Mg. O (O. N. of O = -2), HBr. O 3 (O. N. of O = -2) Exceptions include peroxides where it is -1 H 2 O 2 (O. N. of O = -1)
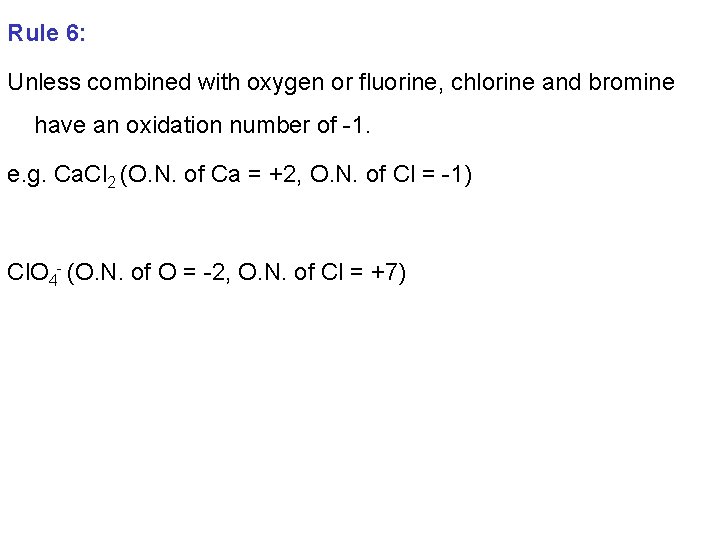
Rule 6: Unless combined with oxygen or fluorine, chlorine and bromine have an oxidation number of -1. e. g. Ca. Cl 2 (O. N. of Ca = +2, O. N. of Cl = -1) Cl. O 4 - (O. N. of O = -2, O. N. of Cl = +7)
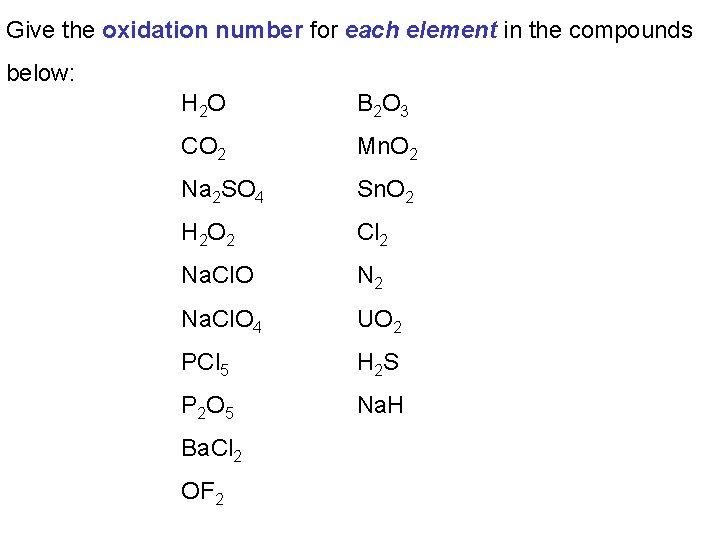
Give the oxidation number for each element in the compounds below: H 2 O B 2 O 3 CO 2 Mn. O 2 Na 2 SO 4 Sn. O 2 H 2 O 2 Cl 2 Na. Cl. O N 2 Na. Cl. O 4 UO 2 PCl 5 H 2 S P 2 O 5 Na. H Ba. Cl 2 OF 2
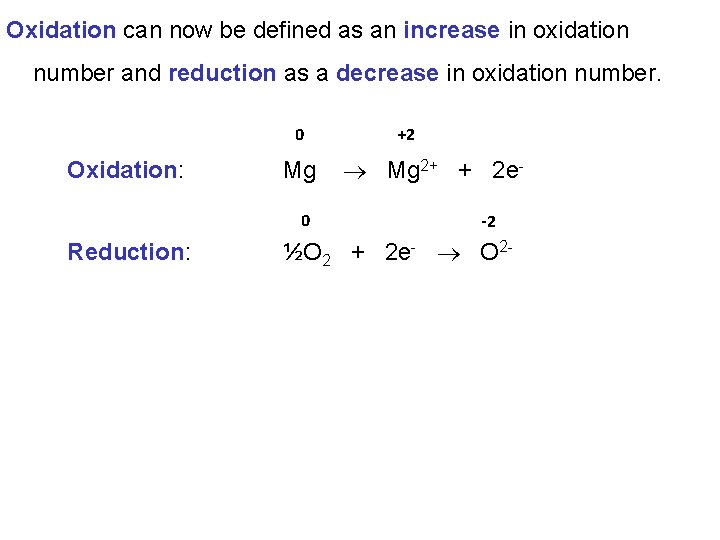
Oxidation can now be defined as an increase in oxidation number and reduction as a decrease in oxidation number. 0 Oxidation: Mg 0 Reduction: +2 Mg 2+ + 2 e-2 ½O 2 + 2 e- O 2 -
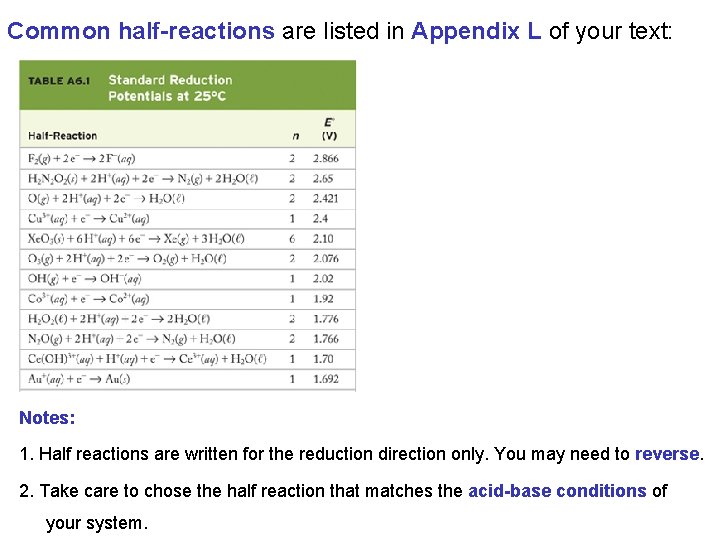
Common half-reactions are listed in Appendix L of your text: Notes: 1. Half reactions are written for the reduction direction only. You may need to reverse. 2. Take care to chose the half reaction that matches the acid-base conditions of your system.
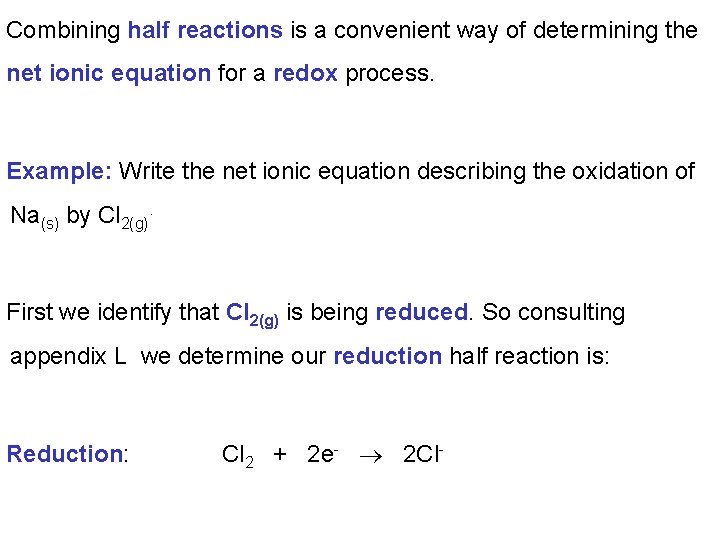
Combining half reactions is a convenient way of determining the net ionic equation for a redox process. Example: Write the net ionic equation describing the oxidation of Na(s) by Cl 2(g). First we identify that Cl 2(g) is being reduced. So consulting appendix L we determine our reduction half reaction is: Reduction: Cl 2 + 2 e- 2 Cl-
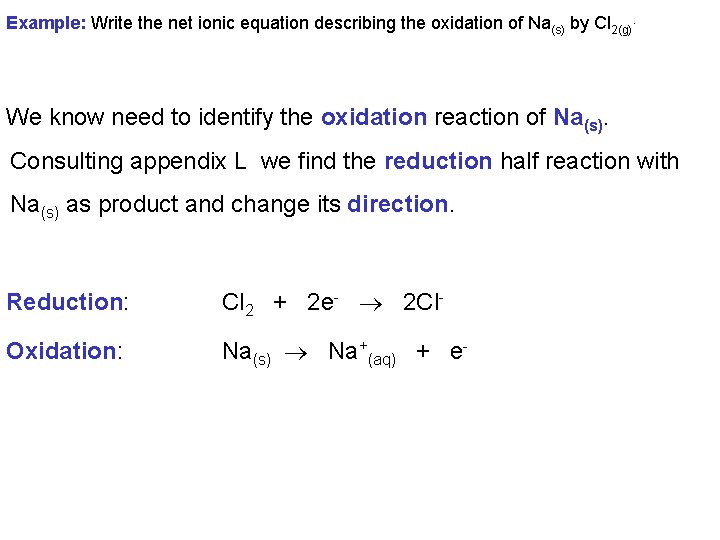
Example: Write the net ionic equation describing the oxidation of Na(s) by Cl 2(g). We know need to identify the oxidation reaction of Na(s). Consulting appendix L we find the reduction half reaction with Na(s) as product and change its direction. Reduction: Cl 2 + 2 e- 2 Cl- Oxidation: Na(s) Na+(aq) + e-
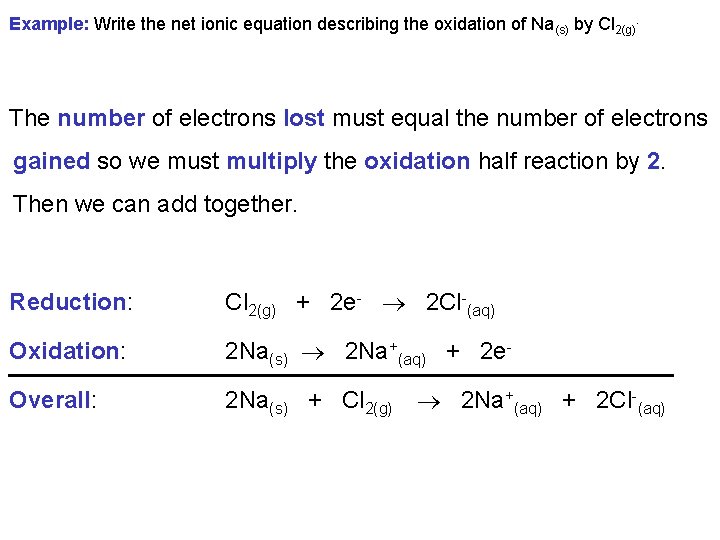
Example: Write the net ionic equation describing the oxidation of Na(s) by Cl 2(g). The number of electrons lost must equal the number of electrons gained so we must multiply the oxidation half reaction by 2. Then we can add together. Reduction: Cl 2(g) + 2 e- 2 Cl-(aq) Oxidation: 2 Na(s) 2 Na+(aq) + 2 e- Overall: 2 Na(s) + Cl 2(g) 2 Na+(aq) + 2 Cl-(aq)
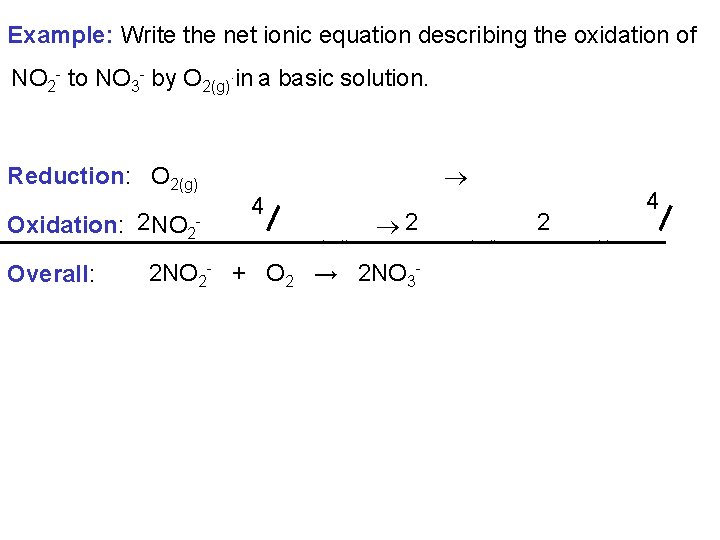
Example: Write the net ionic equation describing the oxidation of NO 2 - to NO 3 - by O 2(g). in a basic solution. Reduction: O 2(g) + 2 H 2 O(l) + 4 e- 4 OH-(aq) 4 4 Oxidation: 2 NO 2 - + 2 OH-(aq) 2 NO 3 -(aq) + 2 H 2 O(l) + 2 e. Overall: 2 NO 2 - + O 2 → 2 NO 3 -
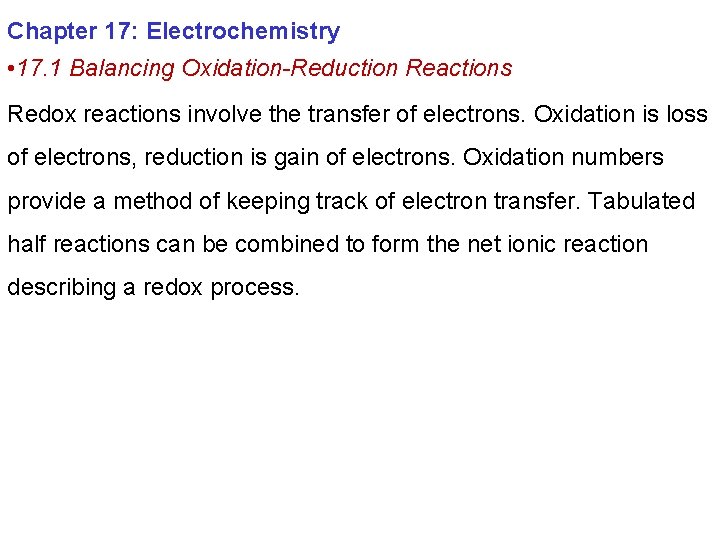
Chapter 17: Electrochemistry • 17. 1 Balancing Oxidation-Reduction Reactions Redox reactions involve the transfer of electrons. Oxidation is loss of electrons, reduction is gain of electrons. Oxidation numbers provide a method of keeping track of electron transfer. Tabulated half reactions can be combined to form the net ionic reaction describing a redox process.
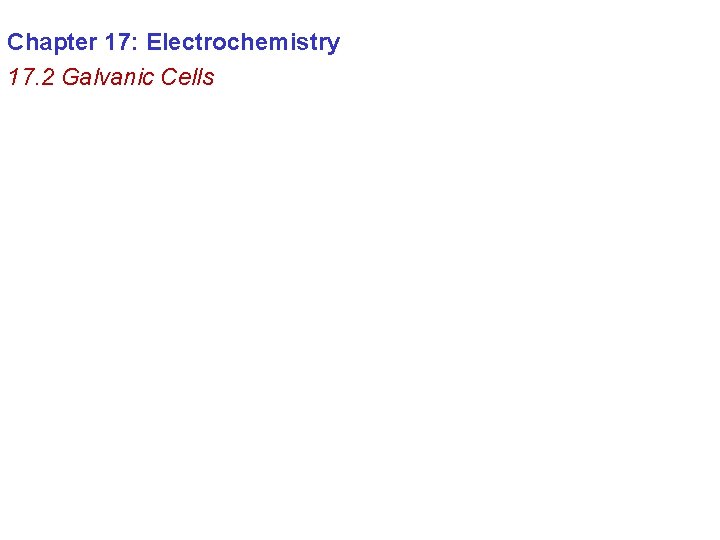
Chapter 17: Electrochemistry 17. 2 Galvanic Cells
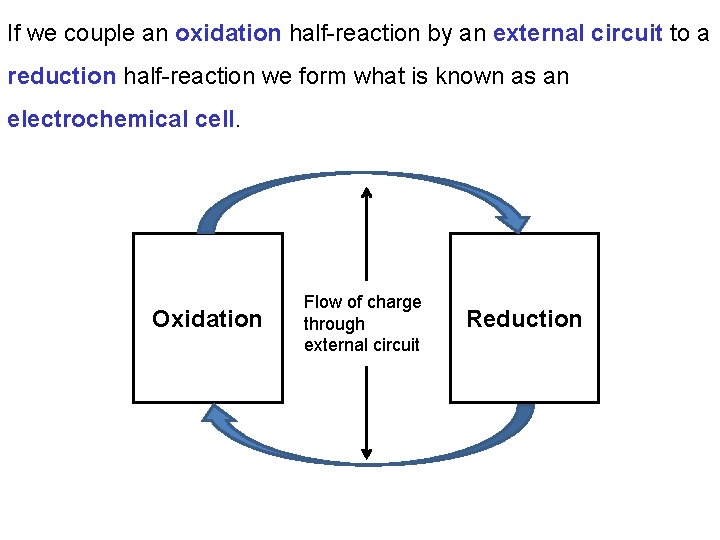
If we couple an oxidation half-reaction by an external circuit to a reduction half-reaction we form what is known as an electrochemical cell. Oxidation Flow of charge through external circuit Reduction
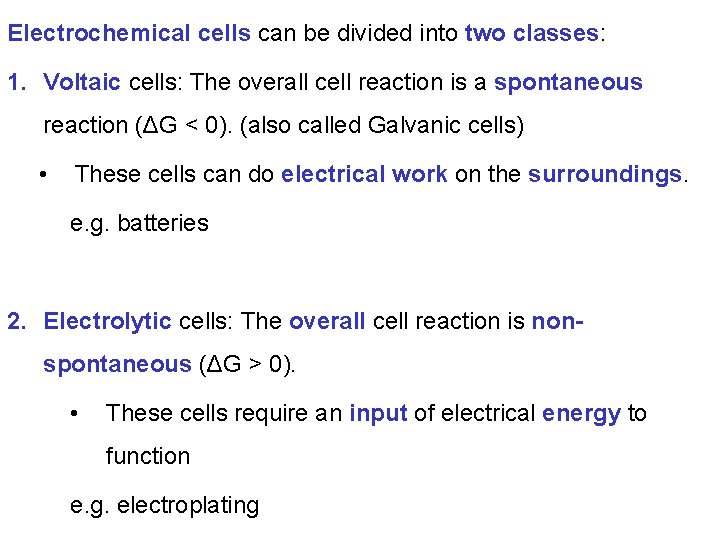
Electrochemical cells can be divided into two classes: 1. Voltaic cells: The overall cell reaction is a spontaneous reaction (ΔG < 0). (also called Galvanic cells) • These cells can do electrical work on the surroundings. e. g. batteries 2. Electrolytic cells: The overall cell reaction is nonspontaneous (ΔG > 0). • These cells require an input of electrical energy to function e. g. electroplating
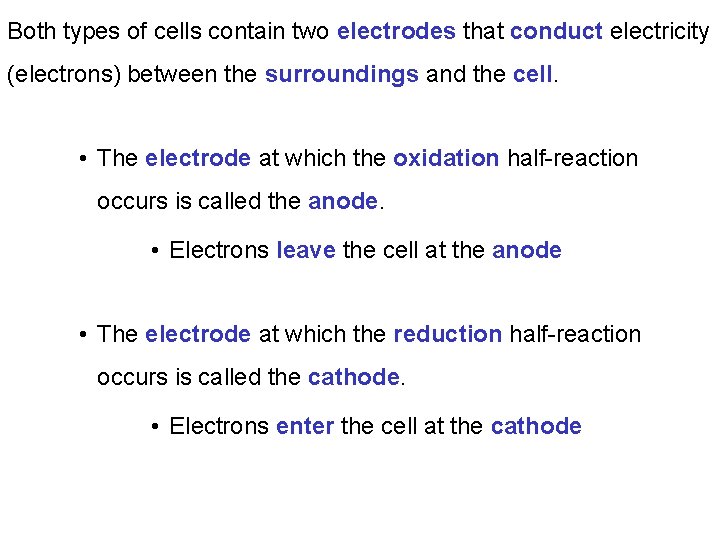
Both types of cells contain two electrodes that conduct electricity (electrons) between the surroundings and the cell. • The electrode at which the oxidation half-reaction occurs is called the anode. • Electrons leave the cell at the anode • The electrode at which the reduction half-reaction occurs is called the cathode. • Electrons enter the cell at the cathode
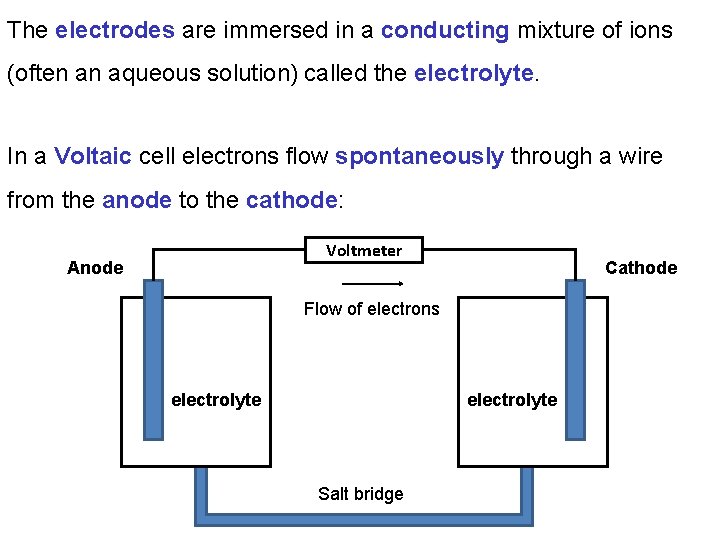
The electrodes are immersed in a conducting mixture of ions (often an aqueous solution) called the electrolyte. In a Voltaic cell electrons flow spontaneously through a wire from the anode to the cathode: Voltmeter Anode Cathode Flow of electrons electrolyte Salt bridge
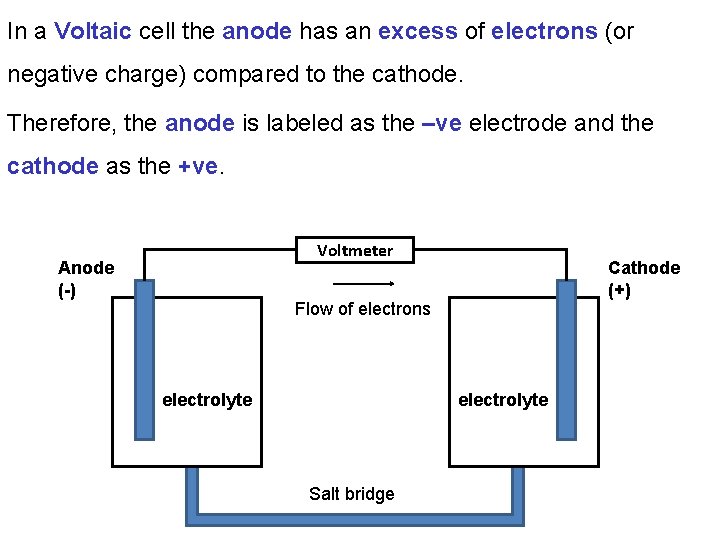
In a Voltaic cell the anode has an excess of electrons (or negative charge) compared to the cathode. Therefore, the anode is labeled as the –ve electrode and the cathode as the +ve. Voltmeter Anode (-) Cathode (+) Flow of electrons electrolyte Salt bridge
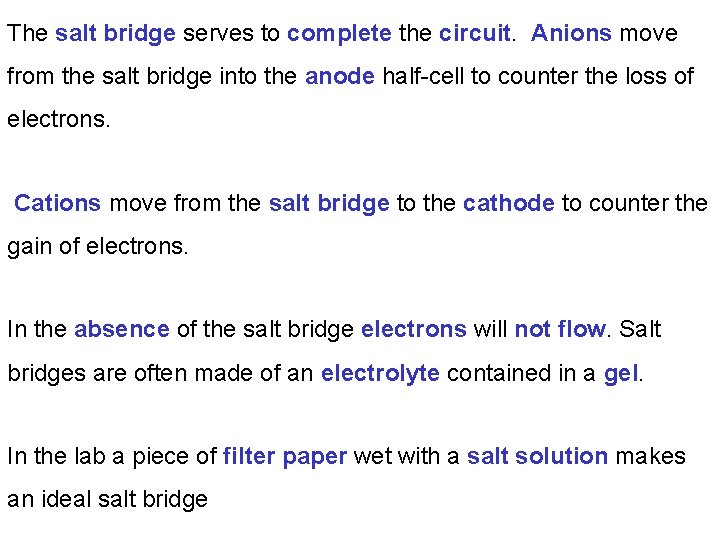
The salt bridge serves to complete the circuit. Anions move from the salt bridge into the anode half-cell to counter the loss of electrons. Cations move from the salt bridge to the cathode to counter the gain of electrons. In the absence of the salt bridge electrons will not flow. Salt bridges are often made of an electrolyte contained in a gel. In the lab a piece of filter paper wet with a salt solution makes an ideal salt bridge
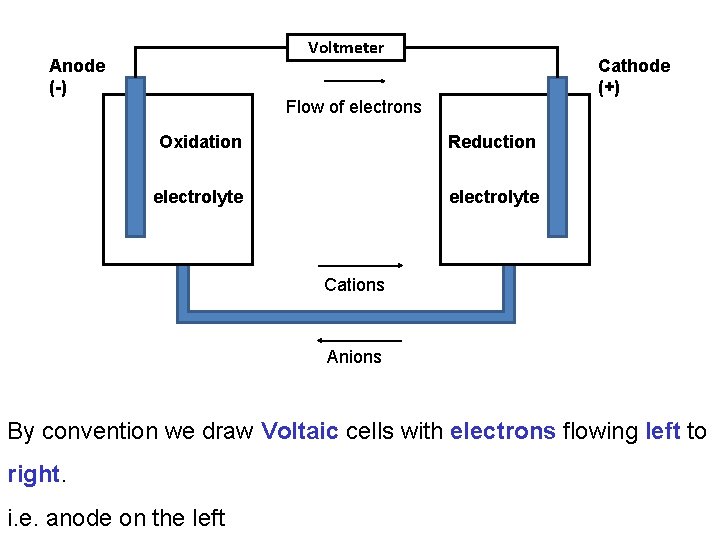
Voltmeter Anode (-) Cathode (+) Flow of electrons Oxidation Reduction electrolyte Cations Anions By convention we draw Voltaic cells with electrons flowing left to right. i. e. anode on the left
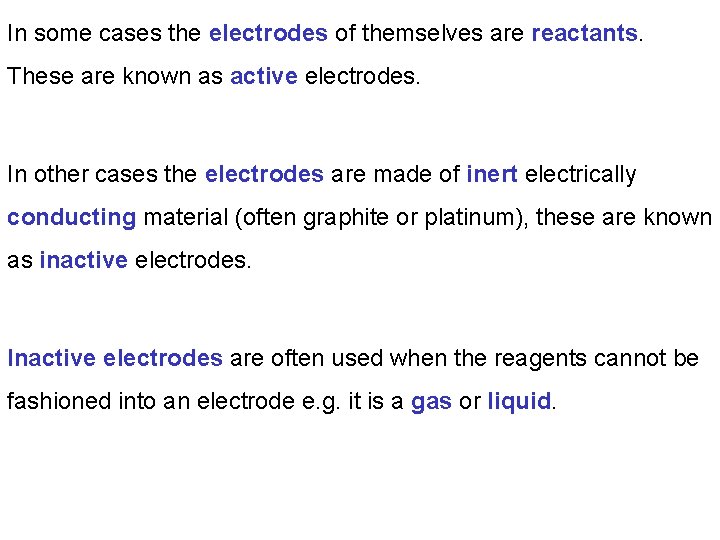
In some cases the electrodes of themselves are reactants. These are known as active electrodes. In other cases the electrodes are made of inert electrically conducting material (often graphite or platinum), these are known as inactive electrodes. Inactive electrodes are often used when the reagents cannot be fashioned into an electrode e. g. it is a gas or liquid.
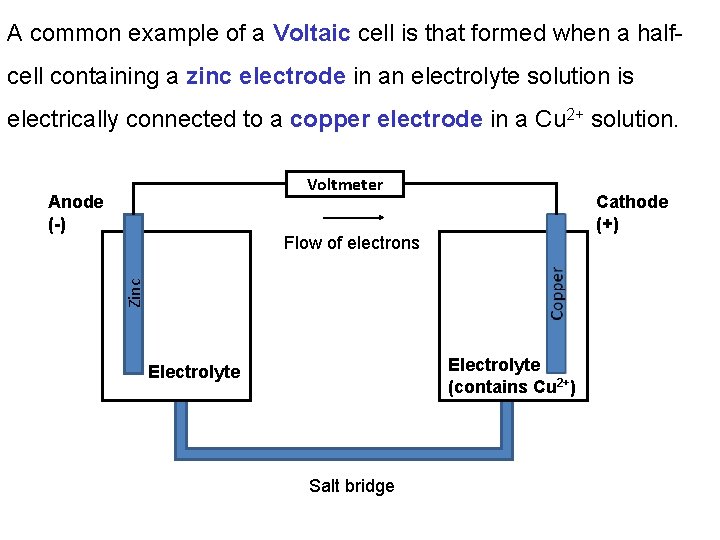
A common example of a Voltaic cell is that formed when a halfcell containing a zinc electrode in an electrolyte solution is electrically connected to a copper electrode in a Cu 2+ solution. Voltmeter Anode (-) Cathode (+) Zinc Flow of electrons Electrolyte (contains Cu 2+) Electrolyte Salt bridge
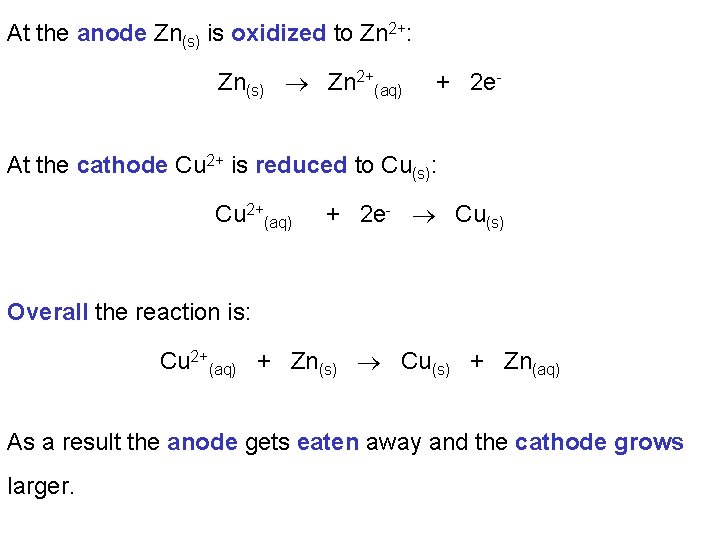
At the anode Zn(s) is oxidized to Zn 2+: Zn(s) Zn 2+(aq) + 2 e- At the cathode Cu 2+ is reduced to Cu(s): Cu 2+(aq) + 2 e- Cu(s) Overall the reaction is: Cu 2+(aq) + Zn(s) Cu(s) + Zn(aq) As a result the anode gets eaten away and the cathode grows larger.
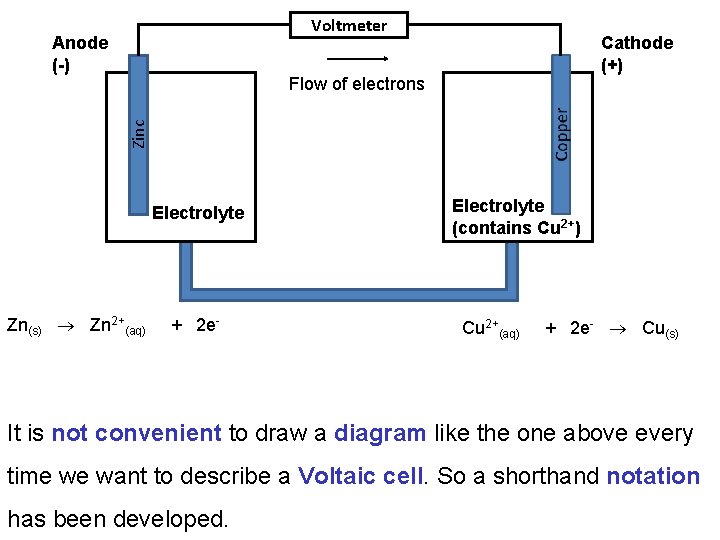
Voltmeter Anode (-) Cathode (+) Zinc Flow of electrons Electrolyte Zn(s) Zn 2+(aq) + 2 e- Electrolyte (contains Cu 2+) Cu 2+(aq) + 2 e- Cu(s) It is not convenient to draw a diagram like the one above every time we want to describe a Voltaic cell. So a shorthand notation has been developed.
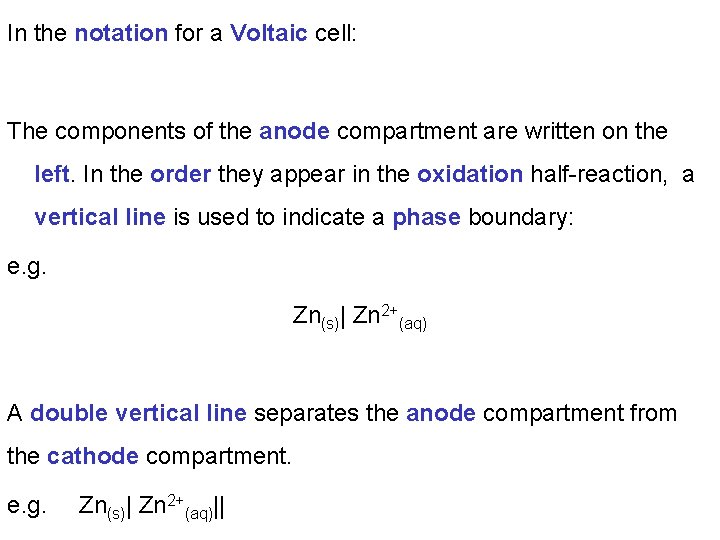
In the notation for a Voltaic cell: The components of the anode compartment are written on the left. In the order they appear in the oxidation half-reaction, a vertical line is used to indicate a phase boundary: e. g. Zn(s)| Zn 2+(aq) A double vertical line separates the anode compartment from the cathode compartment. e. g. Zn(s)| Zn 2+(aq)||
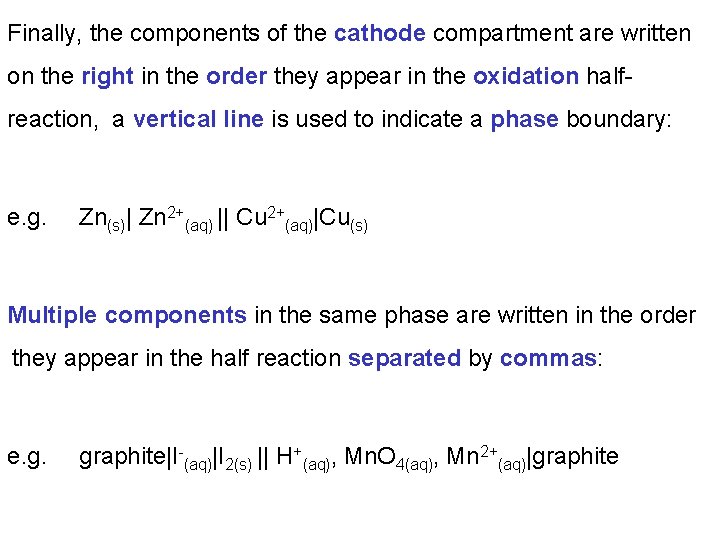
Finally, the components of the cathode compartment are written on the right in the order they appear in the oxidation halfreaction, a vertical line is used to indicate a phase boundary: e. g. Zn(s)| Zn 2+(aq) || Cu 2+(aq)|Cu(s) Multiple components in the same phase are written in the order they appear in the half reaction separated by commas: e. g. graphite|I-(aq)|I 2(s) || H+(aq), Mn. O 4(aq), Mn 2+(aq)|graphite
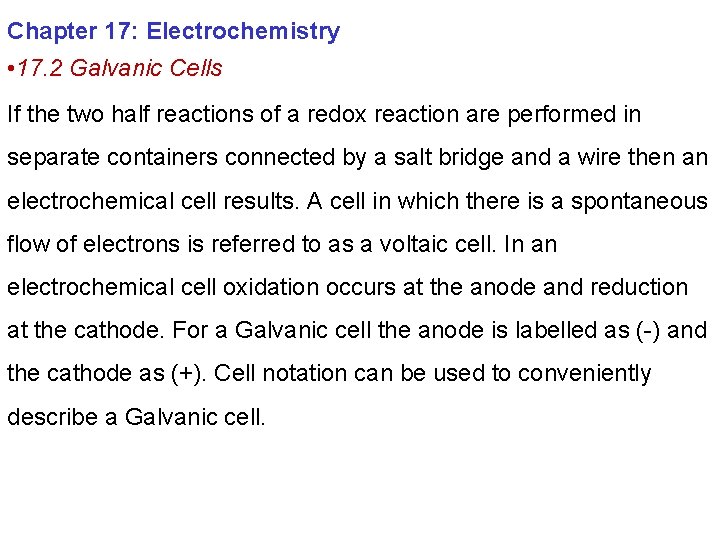
Chapter 17: Electrochemistry • 17. 2 Galvanic Cells If the two half reactions of a redox reaction are performed in separate containers connected by a salt bridge and a wire then an electrochemical cell results. A cell in which there is a spontaneous flow of electrons is referred to as a voltaic cell. In an electrochemical cell oxidation occurs at the anode and reduction at the cathode. For a Galvanic cell the anode is labelled as (-) and the cathode as (+). Cell notation can be used to conveniently describe a Galvanic cell.
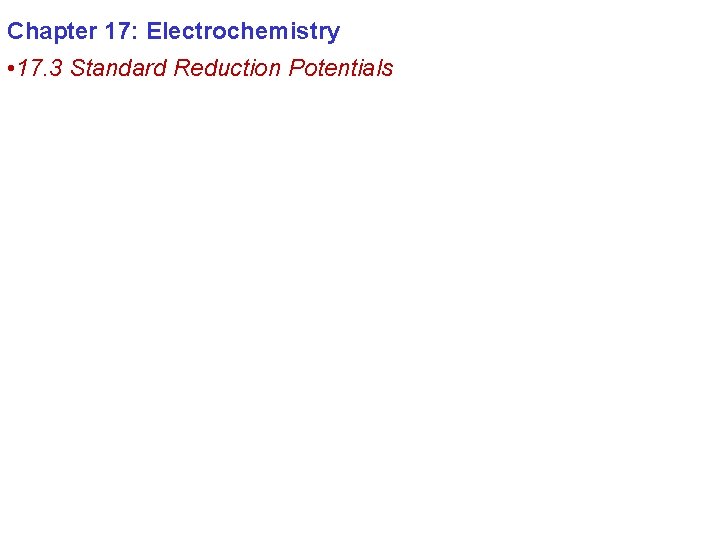
Chapter 17: Electrochemistry • 17. 3 Standard Reduction Potentials
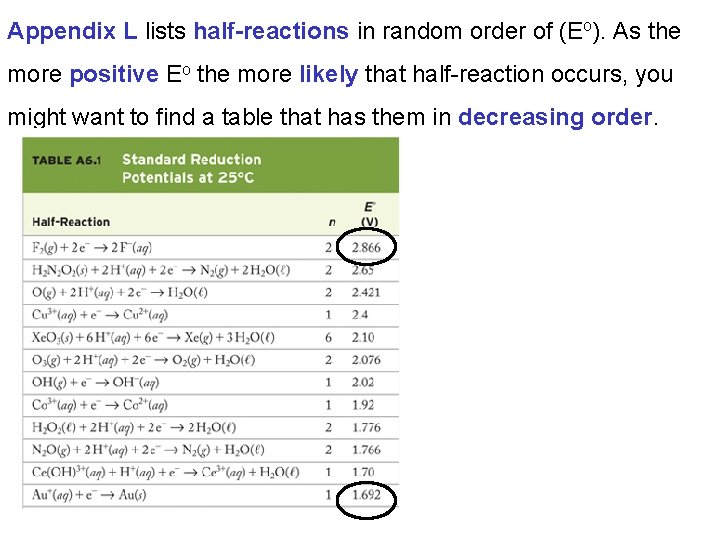
Appendix L lists half-reactions in random order of (Eo). As the more positive Eo the more likely that half-reaction occurs, you might want to find a table that has them in decreasing order.
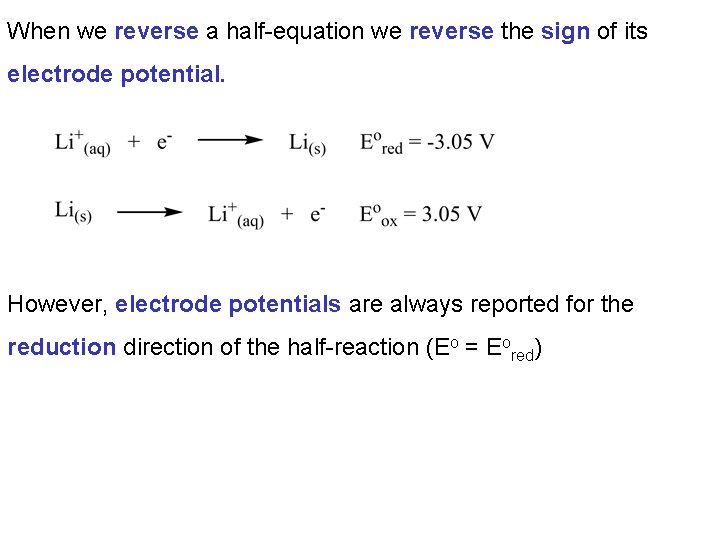
When we reverse a half-equation we reverse the sign of its electrode potential. However, electrode potentials are always reported for the reduction direction of the half-reaction (Eo = Eored)
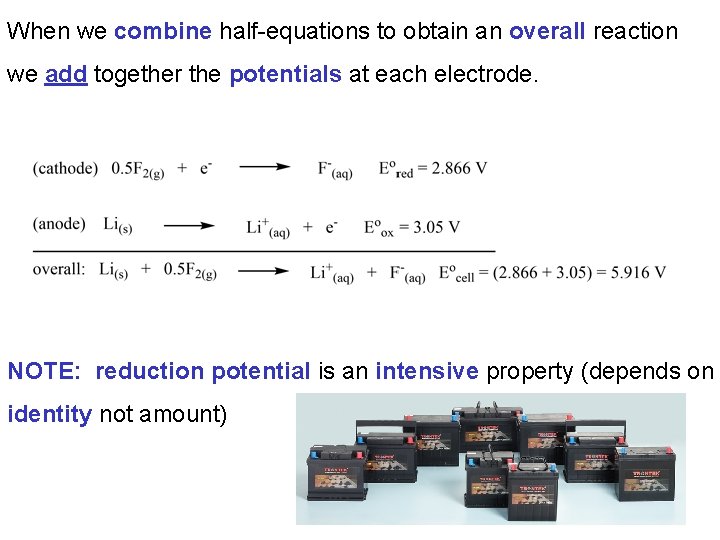
When we combine half-equations to obtain an overall reaction we add together the potentials at each electrode. NOTE: reduction potential is an intensive property (depends on identity not amount)
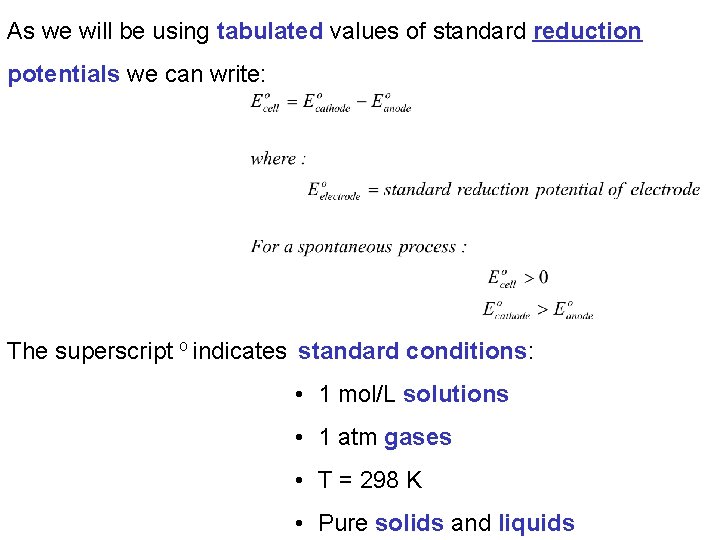
As we will be using tabulated values of standard reduction potentials we can write: The superscript o indicates standard conditions: • 1 mol/L solutions • 1 atm gases • T = 298 K • Pure solids and liquids
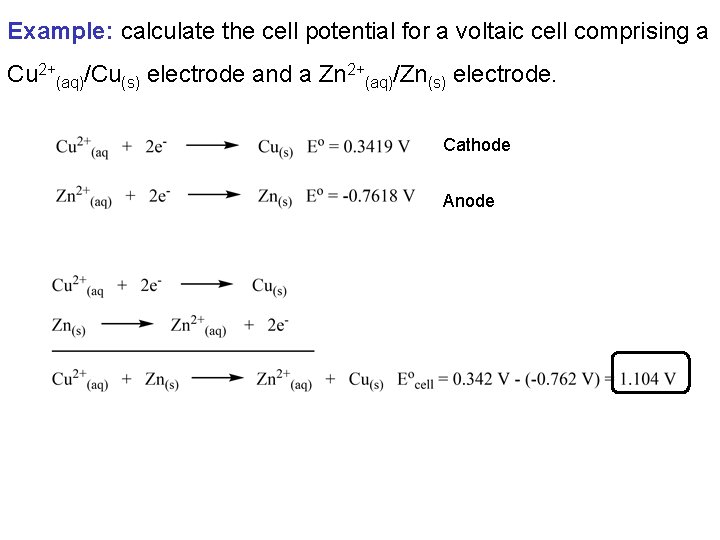
Example: calculate the cell potential for a voltaic cell comprising a Cu 2+(aq)/Cu(s) electrode and a Zn 2+(aq)/Zn(s) electrode. Cathode Anode
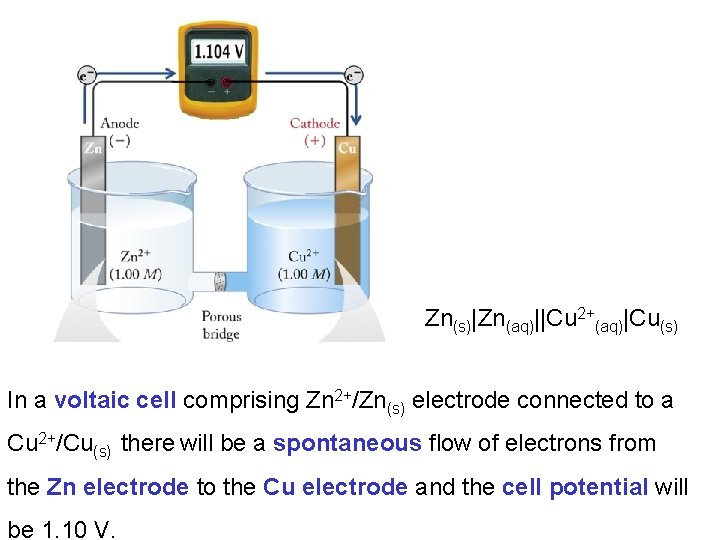
Zn(s)|Zn(aq)||Cu 2+(aq)|Cu(s) In a voltaic cell comprising Zn 2+/Zn(s) electrode connected to a Cu 2+/Cu(s) there will be a spontaneous flow of electrons from the Zn electrode to the Cu electrode and the cell potential will be 1. 10 V.
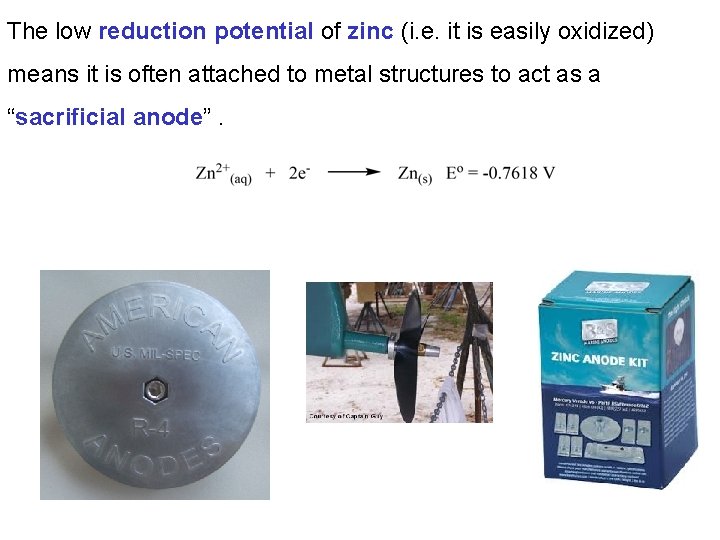
The low reduction potential of zinc (i. e. it is easily oxidized) means it is often attached to metal structures to act as a “sacrificial anode”.
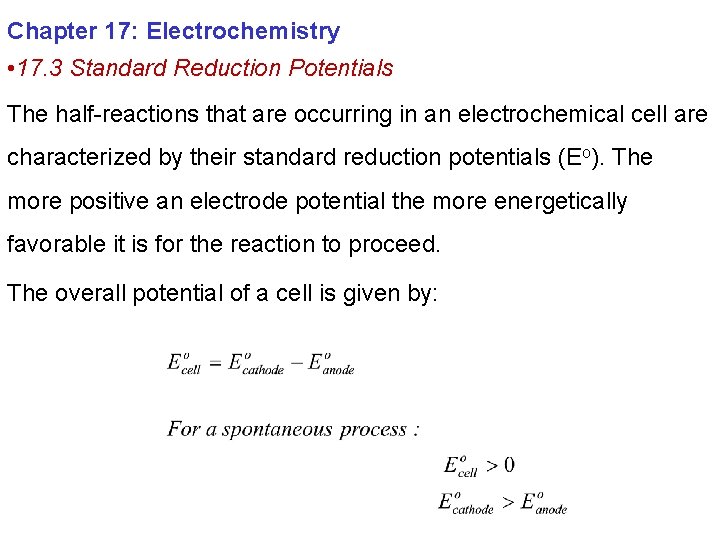
Chapter 17: Electrochemistry • 17. 3 Standard Reduction Potentials The half-reactions that are occurring in an electrochemical cell are characterized by their standard reduction potentials (Eo). The more positive an electrode potential the more energetically favorable it is for the reaction to proceed. The overall potential of a cell is given by:
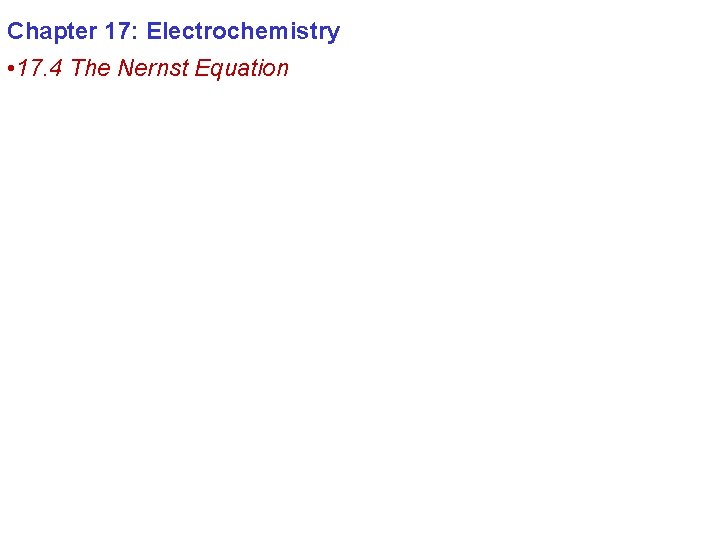
Chapter 17: Electrochemistry • 17. 4 The Nernst Equation
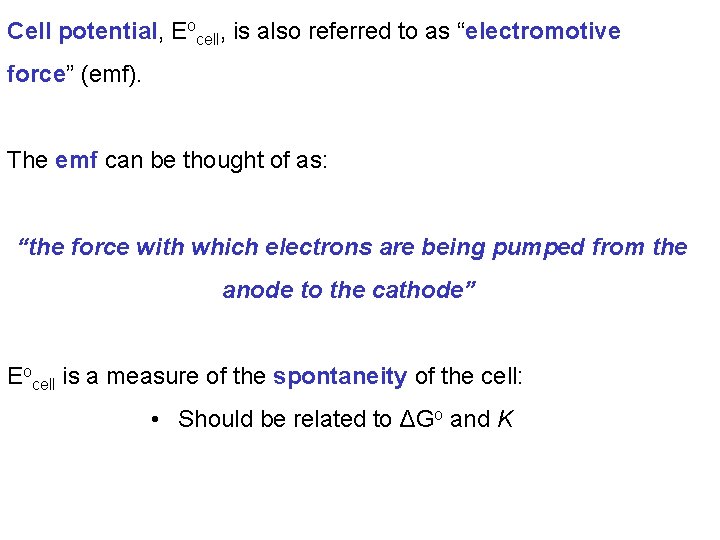
Cell potential, Eocell, is also referred to as “electromotive force” (emf). The emf can be thought of as: “the force with which electrons are being pumped from the anode to the cathode” Eocell is a measure of the spontaneity of the cell: • Should be related to ΔGo and K
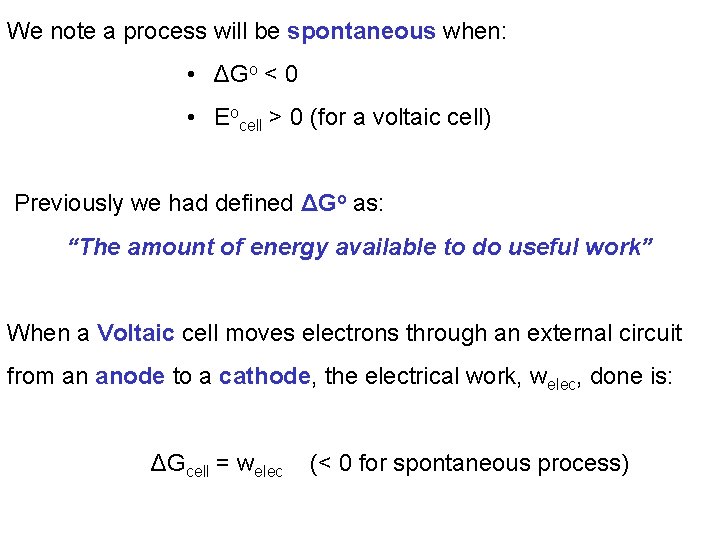
We note a process will be spontaneous when: • ΔGo < 0 • Eocell > 0 (for a voltaic cell) Previously we had defined ΔGo as: “The amount of energy available to do useful work” When a Voltaic cell moves electrons through an external circuit from an anode to a cathode, the electrical work, welec, done is: ΔGcell = welec (< 0 for spontaneous process)
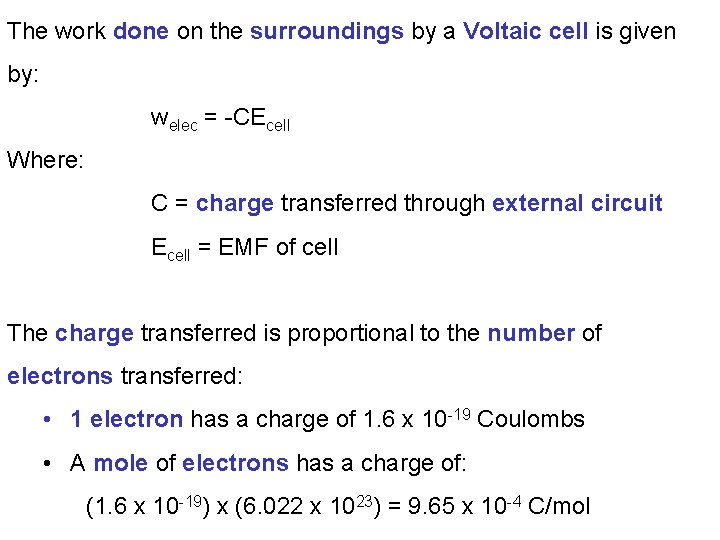
The work done on the surroundings by a Voltaic cell is given by: welec = -CEcell Where: C = charge transferred through external circuit Ecell = EMF of cell The charge transferred is proportional to the number of electrons transferred: • 1 electron has a charge of 1. 6 x 10 -19 Coulombs • A mole of electrons has a charge of: (1. 6 x 10 -19) x (6. 022 x 1023) = 9. 65 x 10 -4 C/mol
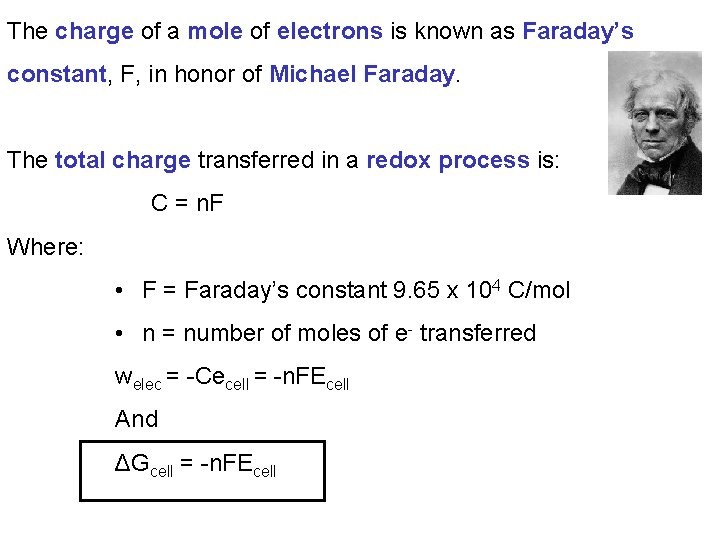
The charge of a mole of electrons is known as Faraday’s constant, F, in honor of Michael Faraday. The total charge transferred in a redox process is: C = n. F Where: • F = Faraday’s constant 9. 65 x 104 C/mol • n = number of moles of e- transferred welec = -Cecell = -n. FEcell And ΔGcell = -n. FEcell
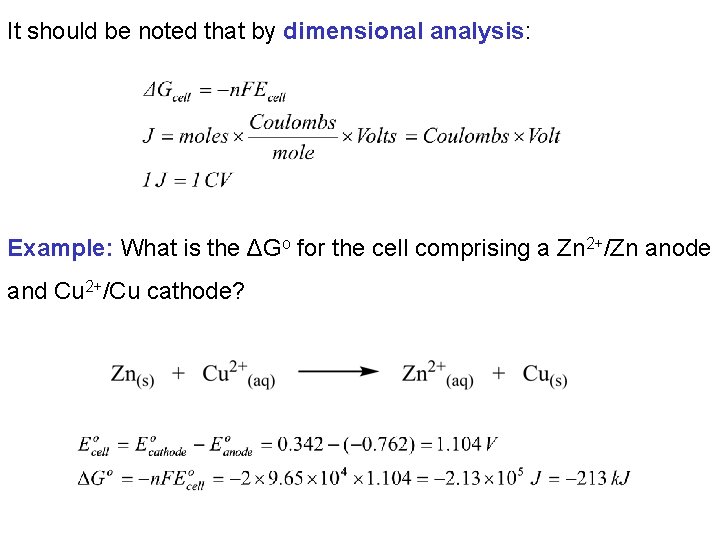
It should be noted that by dimensional analysis: Example: What is the ΔGo for the cell comprising a Zn 2+/Zn anode and Cu 2+/Cu cathode?
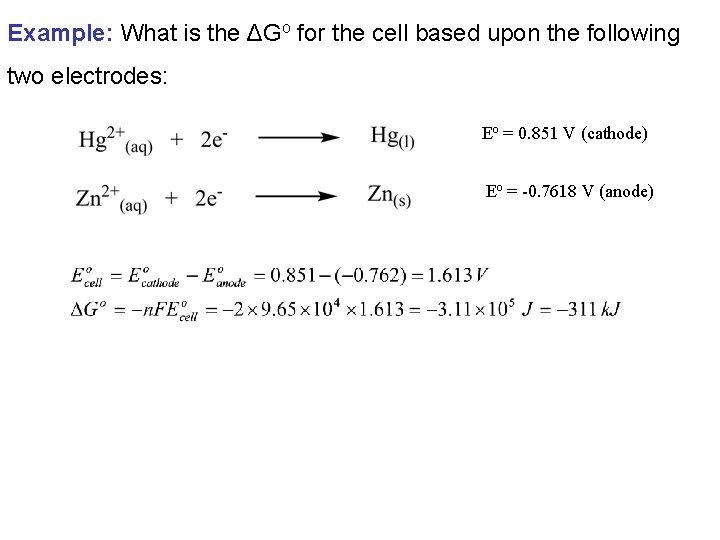
Example: What is the ΔGo for the cell based upon the following two electrodes: Eo = 0. 851 V (cathode) Eo = -0. 7618 V (anode)
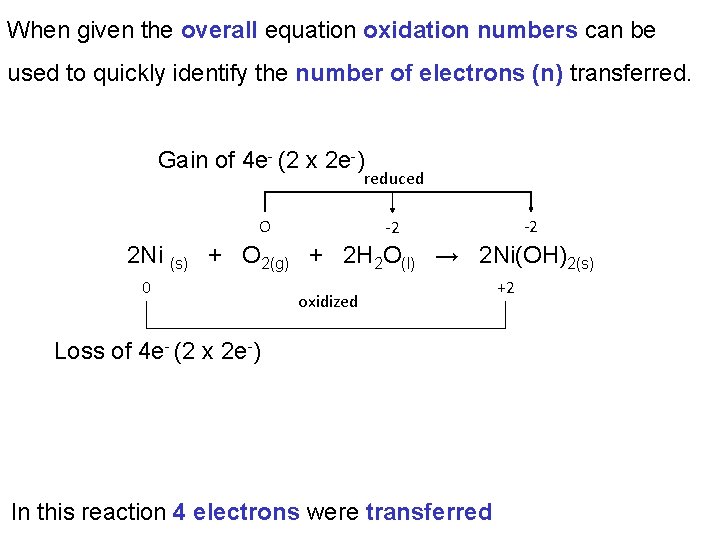
When given the overall equation oxidation numbers can be used to quickly identify the number of electrons (n) transferred. Gain of 4 e- (2 x 2 e-) reduced O -2 -2 2 Ni (s) + O 2(g) + 2 H 2 O(l) → 2 Ni(OH)2(s) 0 oxidized Loss of 4 e- (2 x 2 e-) In this reaction 4 electrons were transferred +2
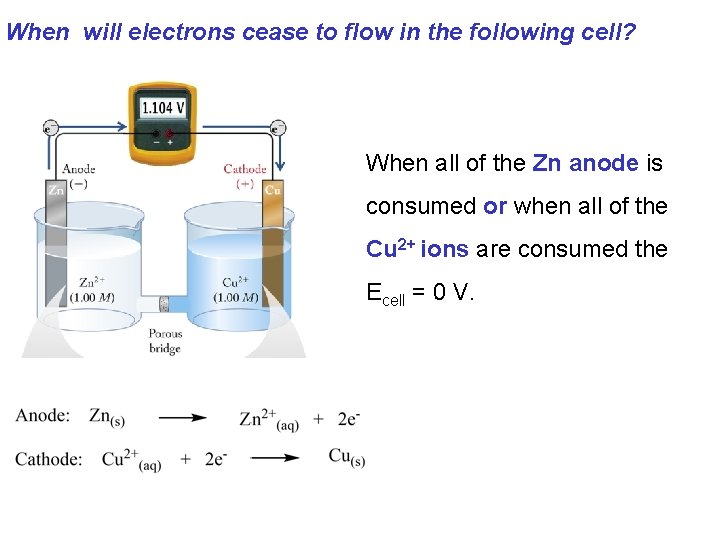
When will electrons cease to flow in the following cell? When all of the Zn anode is consumed or when all of the Cu 2+ ions are consumed the Ecell = 0 V.
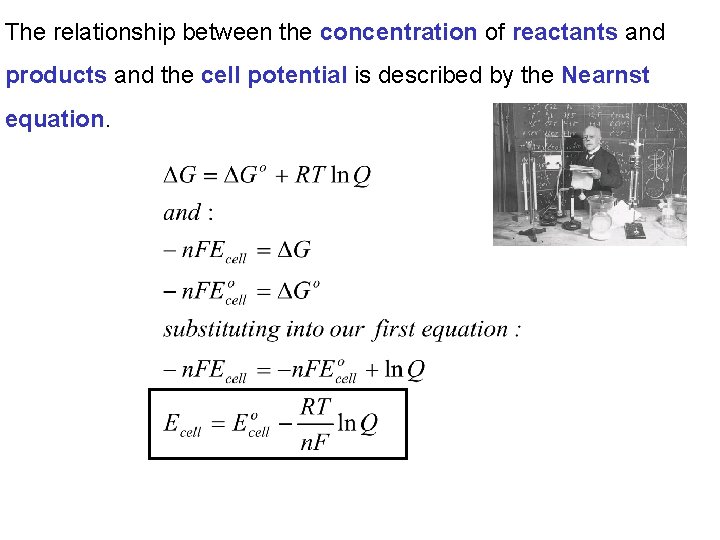
The relationship between the concentration of reactants and products and the cell potential is described by the Nearnst equation.
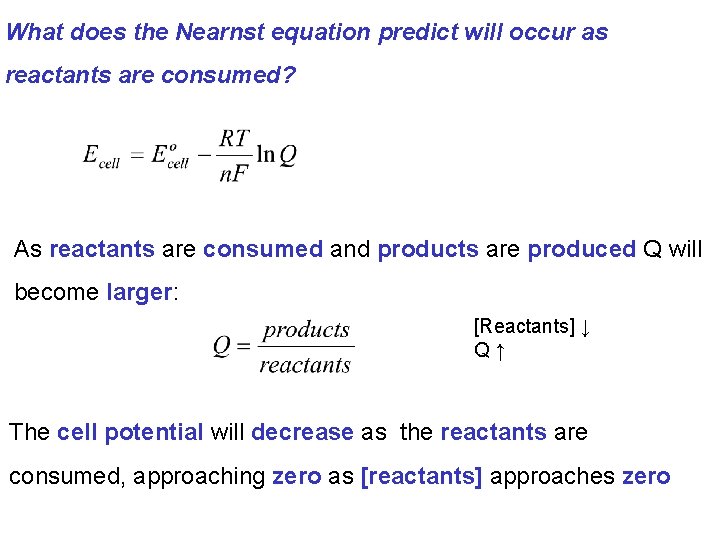
What does the Nearnst equation predict will occur as reactants are consumed? As reactants are consumed and products are produced Q will become larger: [Reactants] ↓ Q↑ The cell potential will decrease as the reactants are consumed, approaching zero as [reactants] approaches zero
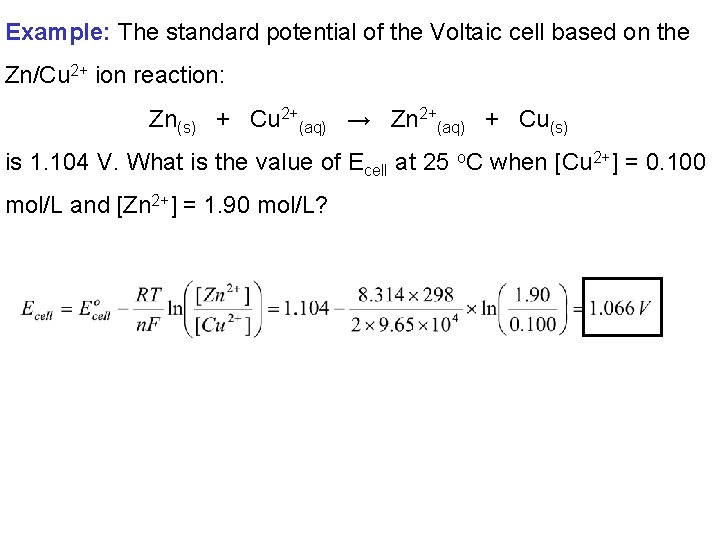
Example: The standard potential of the Voltaic cell based on the Zn/Cu 2+ ion reaction: Zn(s) + Cu 2+(aq) → Zn 2+(aq) + Cu(s) is 1. 104 V. What is the value of Ecell at 25 o. C when [Cu 2+] = 0. 100 mol/L and [Zn 2+] = 1. 90 mol/L?
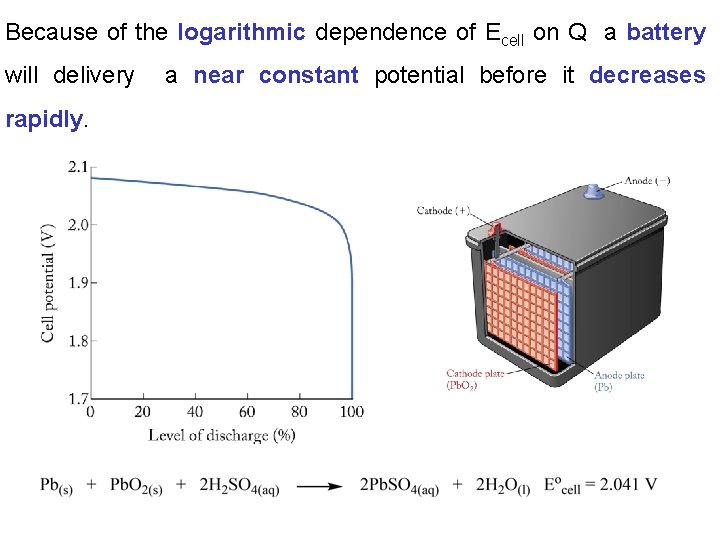
Because of the logarithmic dependence of Ecell on Q a battery will delivery rapidly. a near constant potential before it decreases
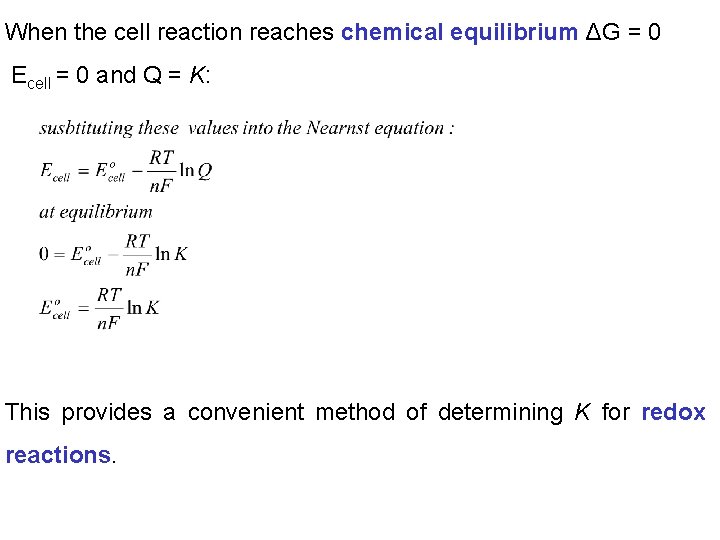
When the cell reaction reaches chemical equilibrium ΔG = 0 Ecell = 0 and Q = K: This provides a convenient method of determining K for redox reactions.
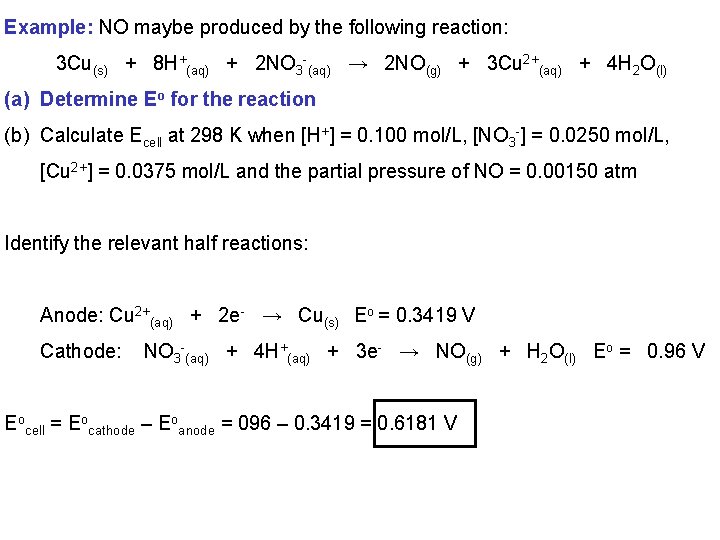
Example: NO maybe produced by the following reaction: 3 Cu(s) + 8 H+(aq) + 2 NO 3 -(aq) → 2 NO(g) + 3 Cu 2+(aq) + 4 H 2 O(l) (a) Determine Eo for the reaction (b) Calculate Ecell at 298 K when [H+] = 0. 100 mol/L, [NO 3 -] = 0. 0250 mol/L, [Cu 2+] = 0. 0375 mol/L and the partial pressure of NO = 0. 00150 atm Identify the relevant half reactions: Anode: Cu 2+(aq) + 2 e- → Cu(s) Eo = 0. 3419 V Cathode: NO 3 -(aq) + 4 H+(aq) + 3 e- → NO(g) + H 2 O(l) Eo = 0. 96 V Eocell = Eocathode – Eoanode = 096 – 0. 3419 = 0. 6181 V
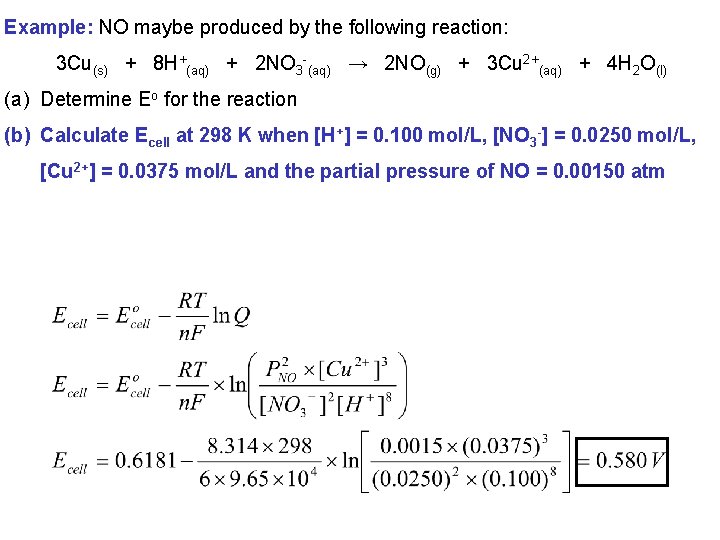
Example: NO maybe produced by the following reaction: 3 Cu(s) + 8 H+(aq) + 2 NO 3 -(aq) → 2 NO(g) + 3 Cu 2+(aq) + 4 H 2 O(l) (a) Determine Eo for the reaction (b) Calculate Ecell at 298 K when [H+] = 0. 100 mol/L, [NO 3 -] = 0. 0250 mol/L, [Cu 2+] = 0. 0375 mol/L and the partial pressure of NO = 0. 00150 atm
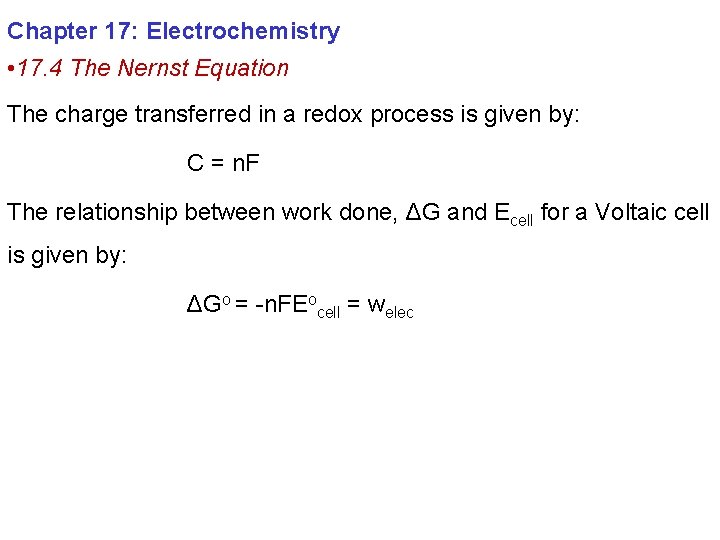
Chapter 17: Electrochemistry • 17. 4 The Nernst Equation The charge transferred in a redox process is given by: C = n. F The relationship between work done, ΔG and Ecell for a Voltaic cell is given by: ΔGo = -n. FEocell = welec
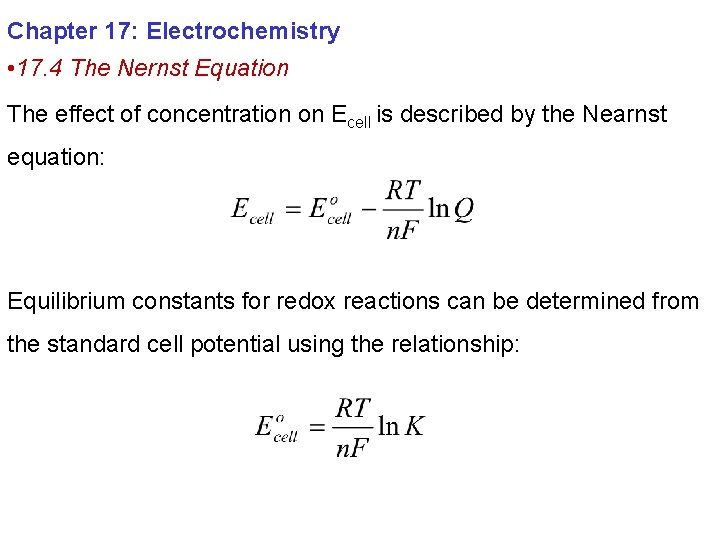
Chapter 17: Electrochemistry • 17. 4 The Nernst Equation The effect of concentration on Ecell is described by the Nearnst equation: Equilibrium constants for redox reactions can be determined from the standard cell potential using the relationship:
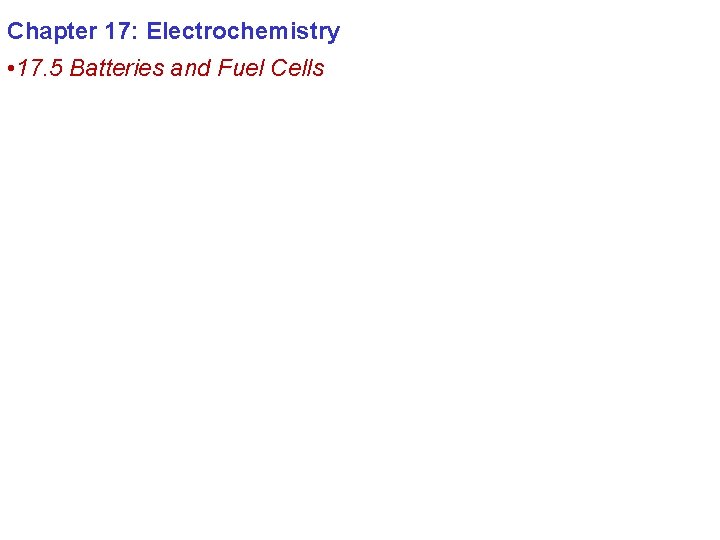
Chapter 17: Electrochemistry • 17. 5 Batteries and Fuel Cells
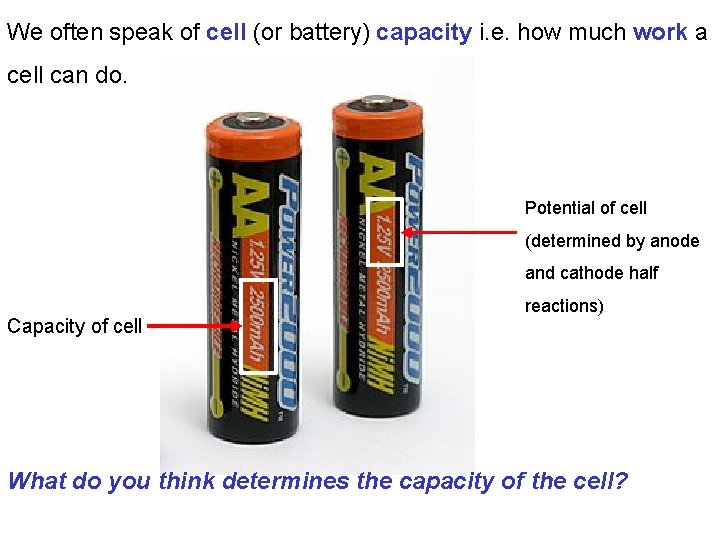
We often speak of cell (or battery) capacity i. e. how much work a cell can do. Potential of cell (determined by anode and cathode half Capacity of cell reactions) What do you think determines the capacity of the cell?
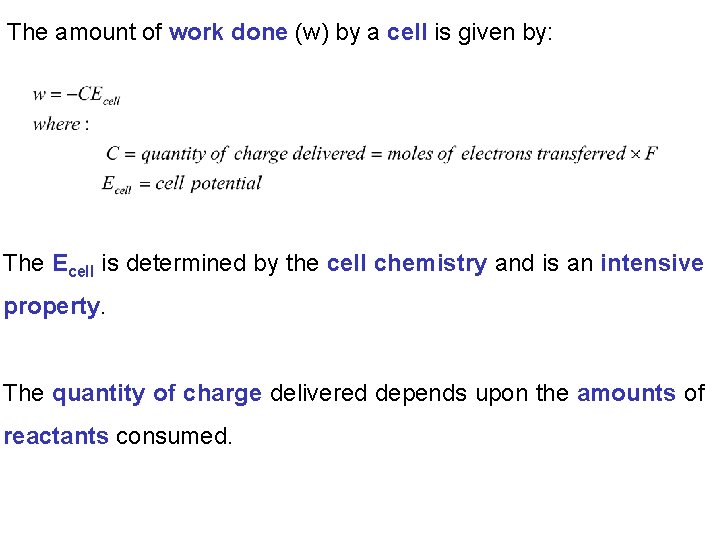
The amount of work done (w) by a cell is given by: The Ecell is determined by the cell chemistry and is an intensive property. The quantity of charge delivered depends upon the amounts of reactants consumed.
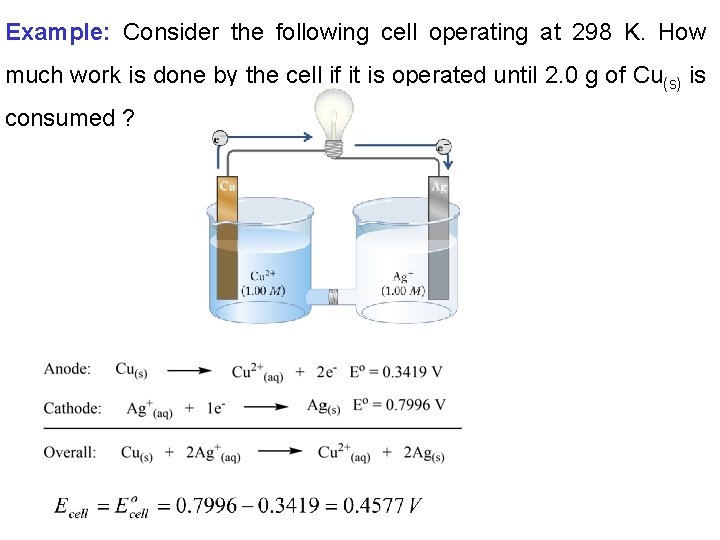
Example: Consider the following cell operating at 298 K. How much work is done by the cell if it is operated until 2. 0 g of Cu(s) is consumed ?
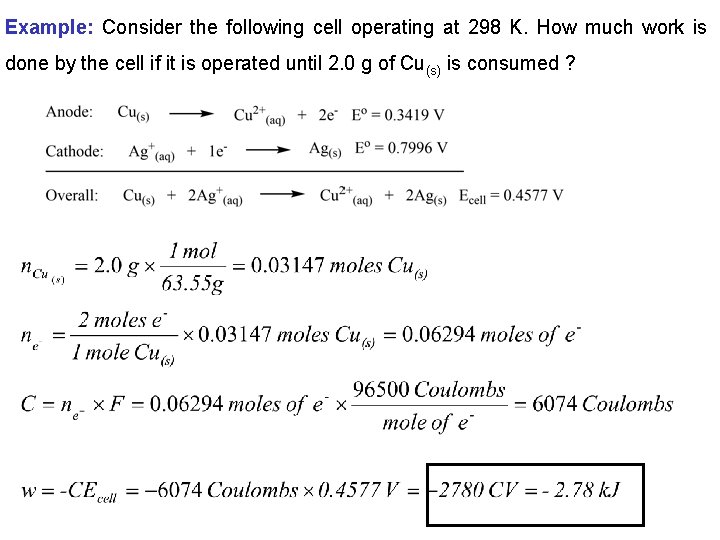
Example: Consider the following cell operating at 298 K. How much work is done by the cell if it is operated until 2. 0 g of Cu(s) is consumed ?
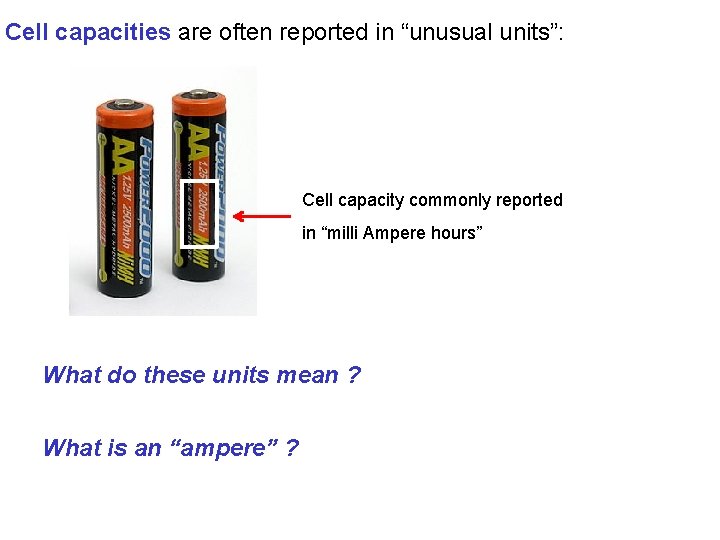
Cell capacities are often reported in “unusual units”: Cell capacity commonly reported in “milli Ampere hours” What do these units mean ? What is an “ampere” ?
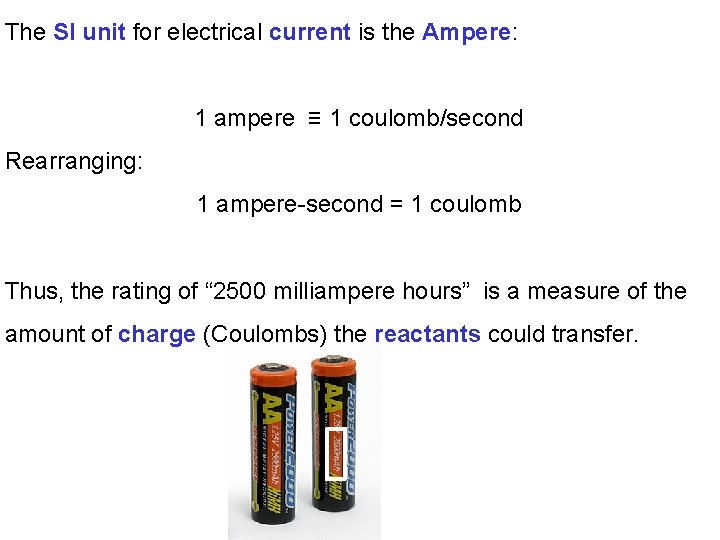
The SI unit for electrical current is the Ampere: 1 ampere ≡ 1 coulomb/second Rearranging: 1 ampere-second = 1 coulomb Thus, the rating of “ 2500 milliampere hours” is a measure of the amount of charge (Coulombs) the reactants could transfer.
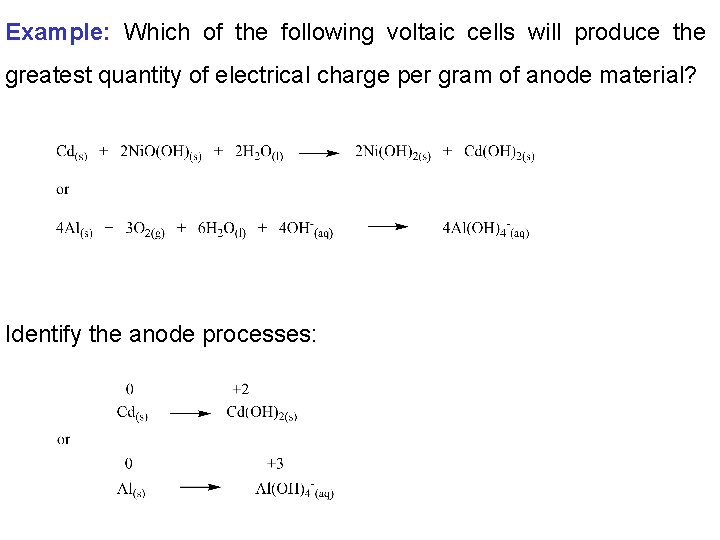
Example: Which of the following voltaic cells will produce the greatest quantity of electrical charge per gram of anode material? Identify the anode processes:
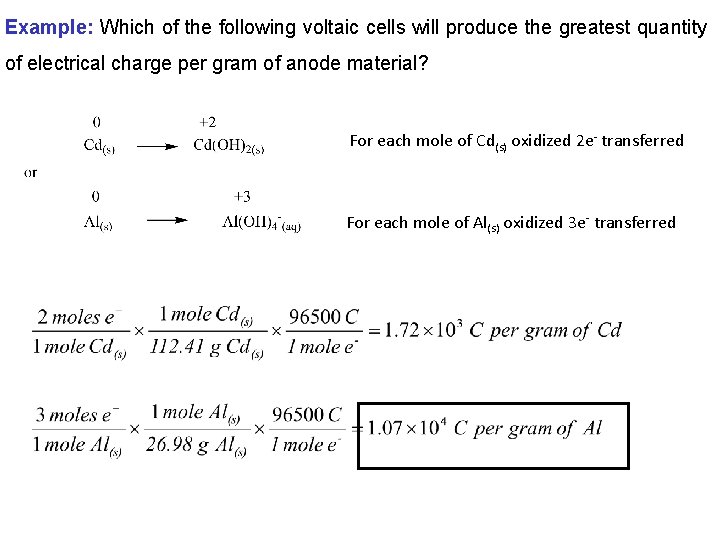
Example: Which of the following voltaic cells will produce the greatest quantity of electrical charge per gram of anode material? For each mole of Cd(s) oxidized 2 e- transferred For each mole of Al(s) oxidized 3 e- transferred
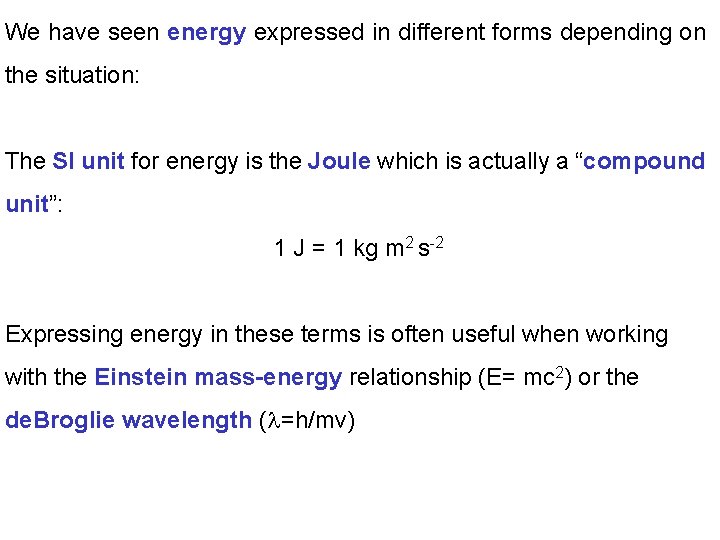
We have seen energy expressed in different forms depending on the situation: The SI unit for energy is the Joule which is actually a “compound unit”: 1 J = 1 kg m 2 s-2 Expressing energy in these terms is often useful when working with the Einstein mass-energy relationship (E= mc 2) or the de. Broglie wavelength (l=h/mv)
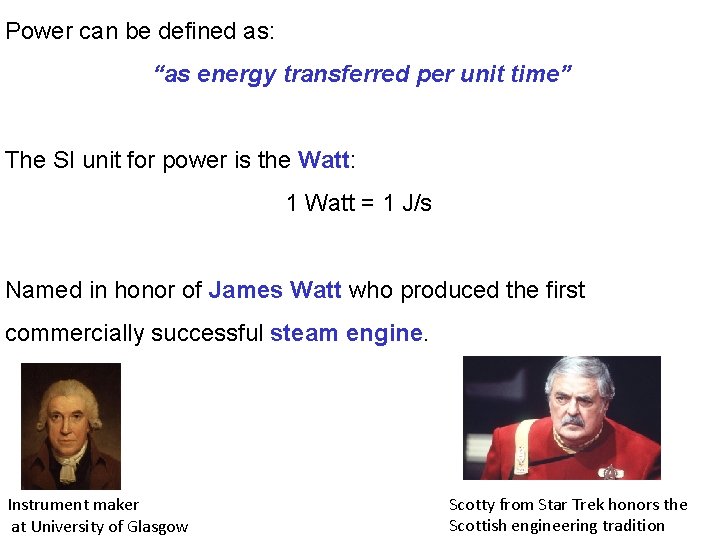
Power can be defined as: “as energy transferred per unit time” The SI unit for power is the Watt: 1 Watt = 1 J/s Named in honor of James Watt who produced the first commercially successful steam engine. Instrument maker at University of Glasgow Scotty from Star Trek honors the Scottish engineering tradition
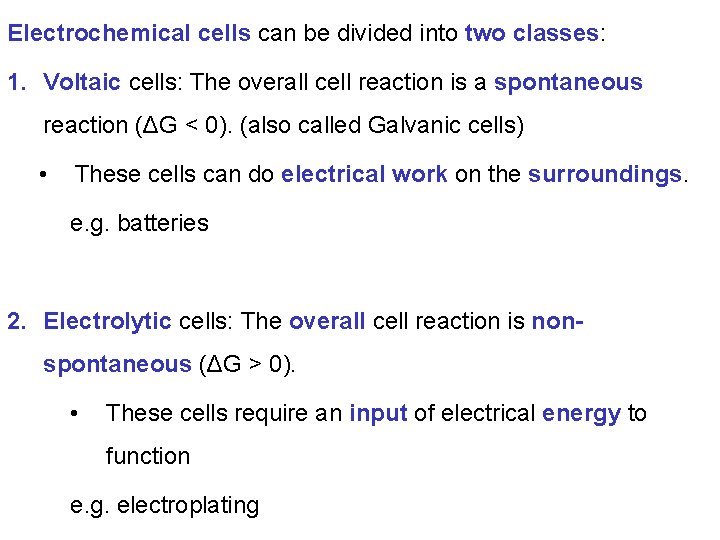
Electrochemical cells can be divided into two classes: 1. Voltaic cells: The overall cell reaction is a spontaneous reaction (ΔG < 0). (also called Galvanic cells) • These cells can do electrical work on the surroundings. e. g. batteries 2. Electrolytic cells: The overall cell reaction is nonspontaneous (ΔG > 0). • These cells require an input of electrical energy to function e. g. electroplating
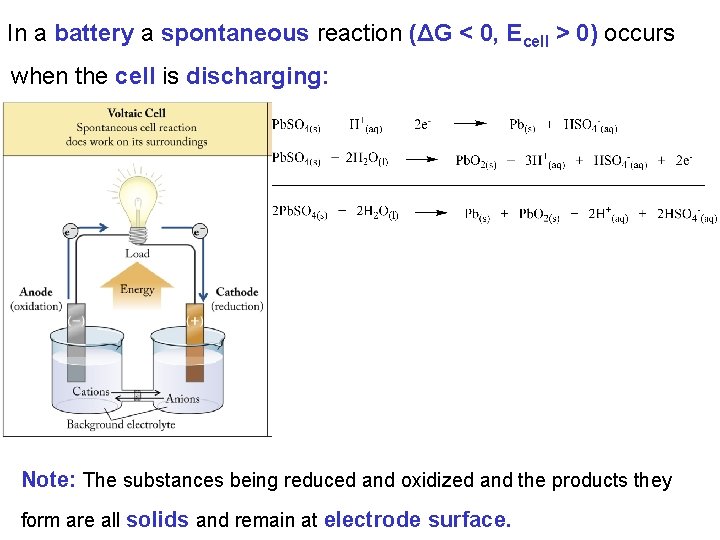
In a battery a spontaneous reaction (ΔG < 0, Ecell > 0) occurs when the cell is discharging: Note: The substances being reduced and oxidized and the products they form are all solids and remain at electrode surface.
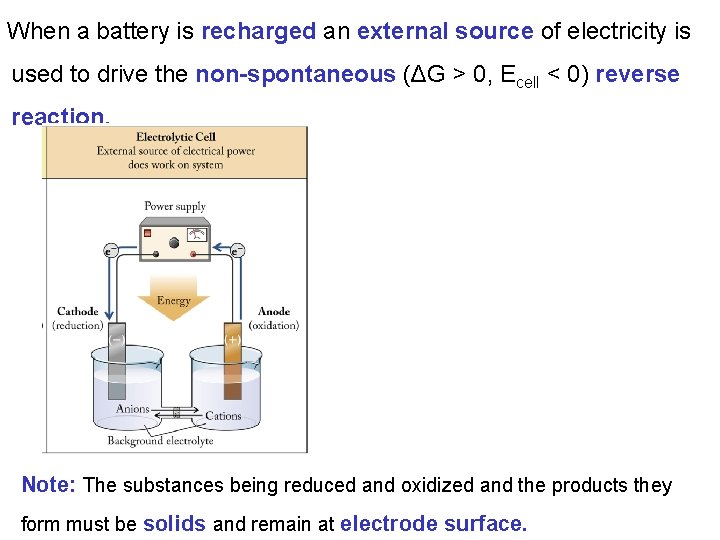
When a battery is recharged an external source of electricity is used to drive the non-spontaneous (ΔG > 0, Ecell < 0) reverse reaction. Note: The substances being reduced and oxidized and the products they form must be solids and remain at electrode surface.
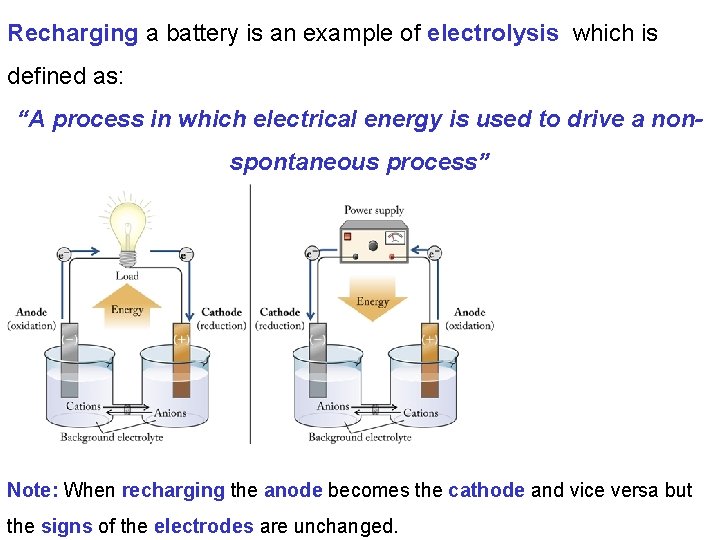
Recharging a battery is an example of electrolysis which is defined as: “A process in which electrical energy is used to drive a nonspontaneous process” Note: When recharging the anode becomes the cathode and vice versa but the signs of the electrodes are unchanged.
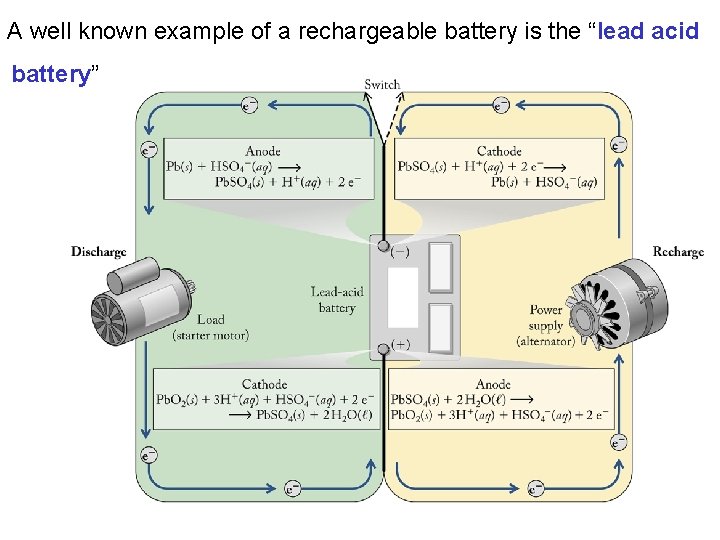
A well known example of a rechargeable battery is the “lead acid battery”
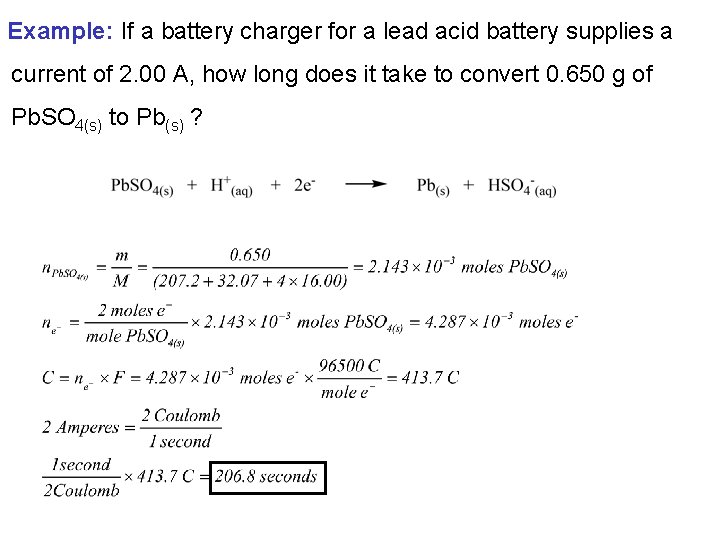
Example: If a battery charger for a lead acid battery supplies a current of 2. 00 A, how long does it take to convert 0. 650 g of Pb. SO 4(s) to Pb(s) ?
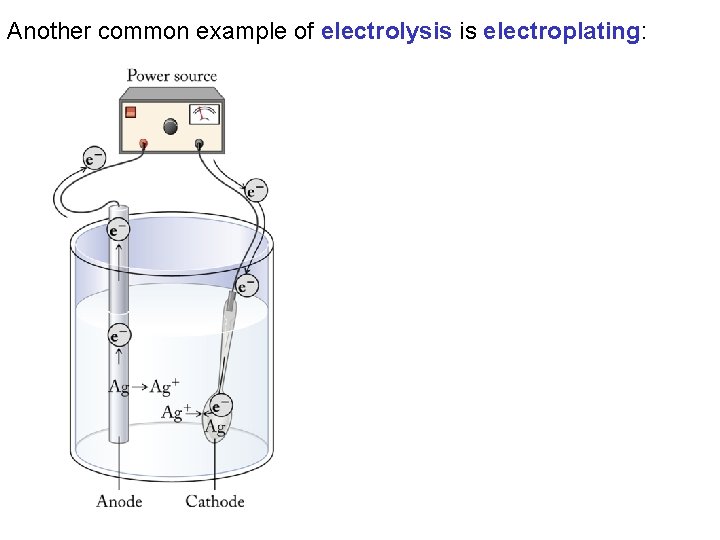
Another common example of electrolysis is electroplating:
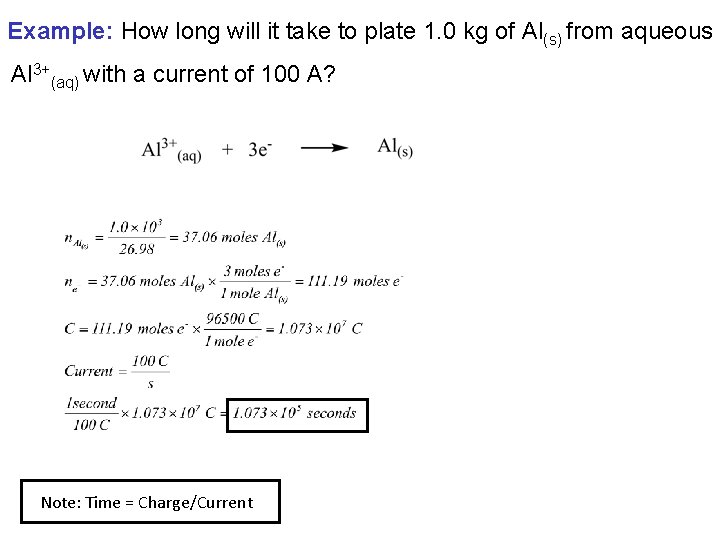
Example: How long will it take to plate 1. 0 kg of Al(s) from aqueous Al 3+(aq) with a current of 100 A? Note: Time = Charge/Current
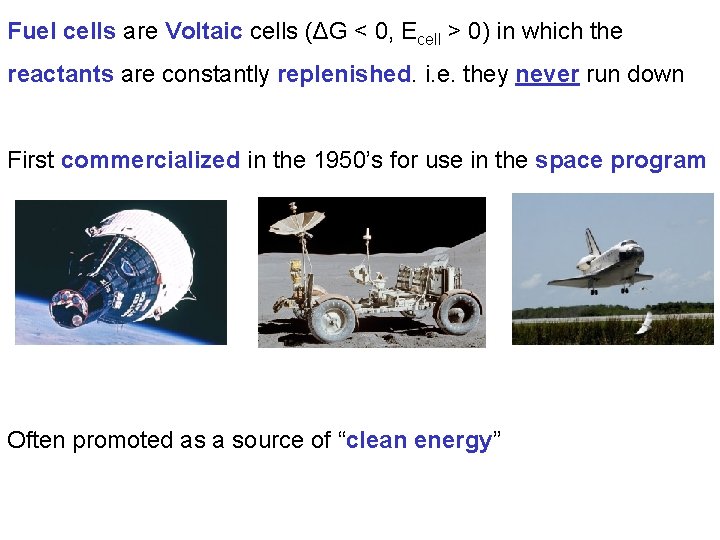
Fuel cells are Voltaic cells (ΔG < 0, Ecell > 0) in which the reactants are constantly replenished. i. e. they never run down First commercialized in the 1950’s for use in the space program Often promoted as a source of “clean energy”
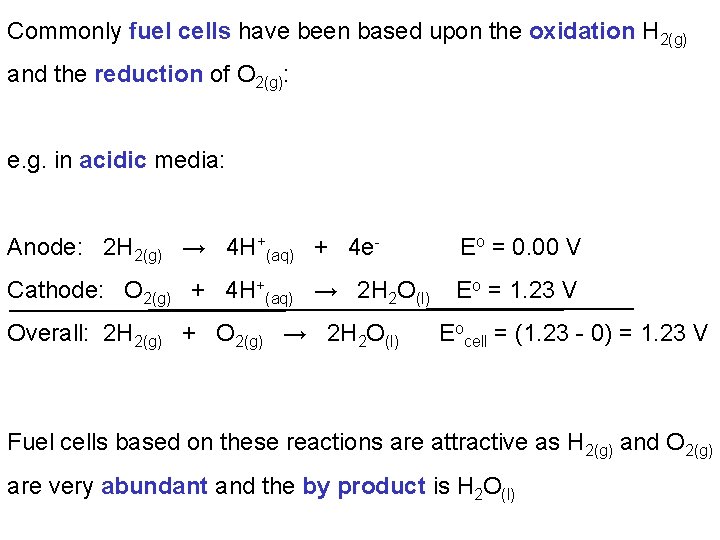
Commonly fuel cells have been based upon the oxidation H 2(g) and the reduction of O 2(g): e. g. in acidic media: Anode: 2 H 2(g) → 4 H+(aq) + 4 e- Eo = 0. 00 V Cathode: O 2(g) + 4 H+(aq) → 2 H 2 O(l) Eo = 1. 23 V Overall: 2 H 2(g) + O 2(g) → 2 H 2 O(l) Eocell = (1. 23 - 0) = 1. 23 V Fuel cells based on these reactions are attractive as H 2(g) and O 2(g) are very abundant and the by product is H 2 O(l)
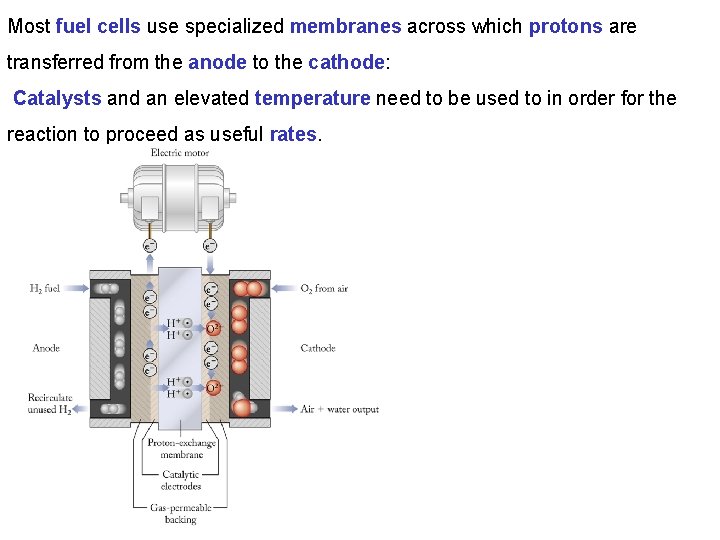
Most fuel cells use specialized membranes across which protons are transferred from the anode to the cathode: Catalysts and an elevated temperature need to be used to in order for the reaction to proceed as useful rates.
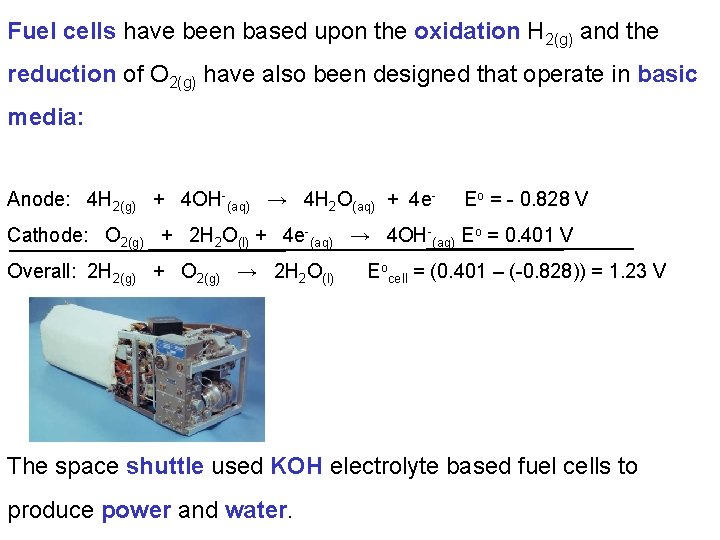
Fuel cells have been based upon the oxidation H 2(g) and the reduction of O 2(g) have also been designed that operate in basic media: Anode: 4 H 2(g) + 4 OH-(aq) → 4 H 2 O(aq) + 4 e- Eo = - 0. 828 V Cathode: O 2(g) + 2 H 2 O(l) + 4 e-(aq) → 4 OH-(aq) Eo = 0. 401 V Overall: 2 H 2(g) + O 2(g) → 2 H 2 O(l) Eocell = (0. 401 – (-0. 828)) = 1. 23 V The space shuttle used KOH electrolyte based fuel cells to produce power and water.
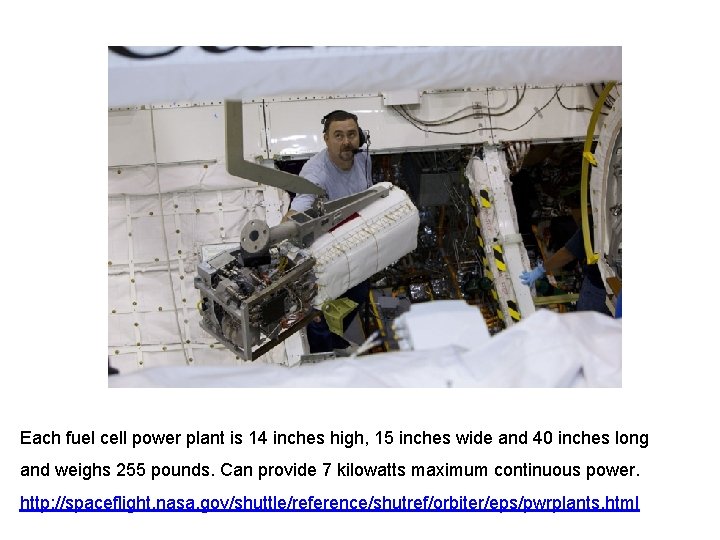
Each fuel cell power plant is 14 inches high, 15 inches wide and 40 inches long and weighs 255 pounds. Can provide 7 kilowatts maximum continuous power. http: //spaceflight. nasa. gov/shuttle/reference/shutref/orbiter/eps/pwrplants. html
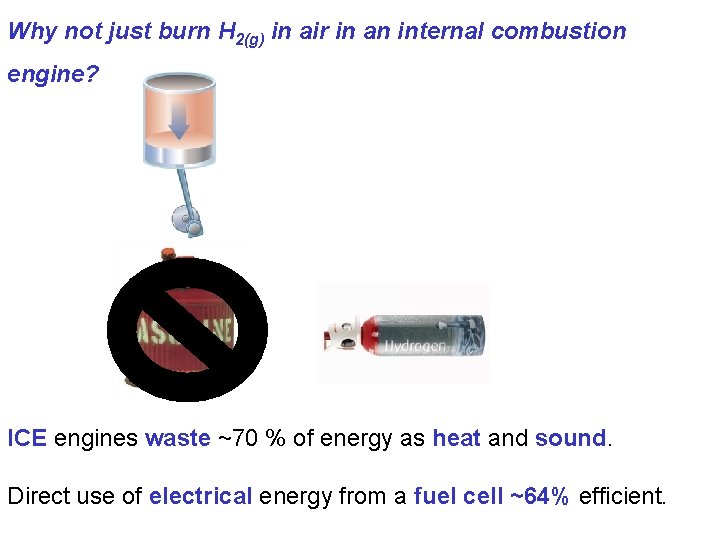
Why not just burn H 2(g) in air in an internal combustion engine? ICE engines waste ~70 % of energy as heat and sound. Direct use of electrical energy from a fuel cell ~64% efficient.
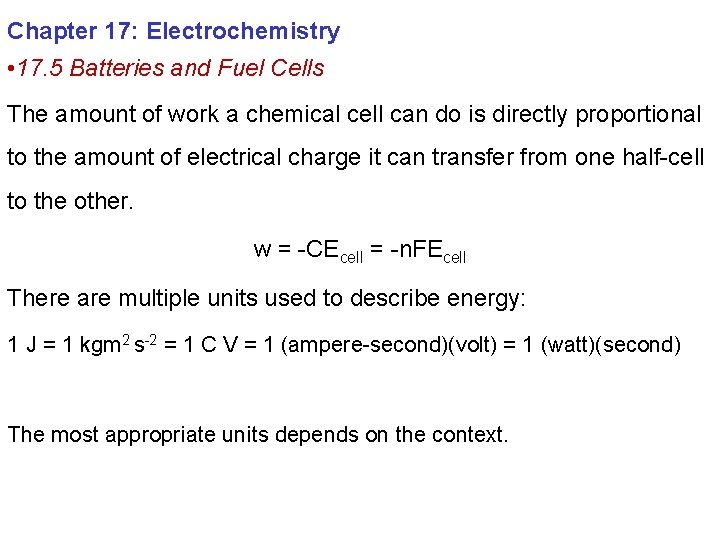
Chapter 17: Electrochemistry • 17. 5 Batteries and Fuel Cells The amount of work a chemical cell can do is directly proportional to the amount of electrical charge it can transfer from one half-cell to the other. w = -CEcell = -n. FEcell There are multiple units used to describe energy: 1 J = 1 kgm 2 s-2 = 1 C V = 1 (ampere-second)(volt) = 1 (watt)(second) The most appropriate units depends on the context.
Ap chemistry chapter 18 electrochemistry test
Danielle cell
What is electrochemistry in chemistry
What is electrochemistry in chemistry
Ap chemistry ced
Introduction to electrochemistry
Ap chem electrochemistry review
Cell chapter 21
Electrolytic cell
Electrons flow from anode to cathode
Pasal 163 is
Pasal 163 is
Apa itu pluralisme
Pasal 163 is
Pasal 163 is
Ent 163
Ent.163
Ent 163
Ent163
Ent163
Ent 163
163 mk
Mc163com
Www.nablwp.qci
An energy source forces a constant current of 2a
Ent.163
Ent.163
Salvacin
Doctrine and covenants 163
163 ent
Ent 163
Cs 121 uci
State finance law 163
Gratido
Design approach
Math 163 ccbc
Faraday's constant electrochemistry
Determination of transport number by hittorf method pdf
Electrochemistry tutorial
What is e in nernst equation
Electrochemistry balancing equations
Electrochemistry stoichiometry
Khan academy balancing equations
Aee cd20f
Balancing complex redox reactions
Cell reaction in electrochemistry
Ecell formula
Interfacial method in electrochemistry
Electrolysis table
Fundamentals of electrochemistry
What is electrochemistry
Cell notation
Diagonal rule electrochemistry
Polarization in electrochemistry
Electrochemistry lesson plan
Branches of electrochemistry
Mass transport electrochemistry
Electroanalytical techniques
Fundamentals of electrochemistry
Electrochemistry
Diagonal rule electrochemistry
Coupon technology
Electrochemistry balancing equations
Whats electrochemistry
Red cat electrochemistry
Cell reaction
Define concentration cells
Electrochemistry
Planos en cinematografia
Where did general lee surrender to general grant?
General chemistry with qualitative analysis
General chemistry thermochemistry
General chemistry nomenclature
General chemistry 2
General chemistry ders notları
General chemistry
General chemistry 1 stoichiometry
General chemistry
General chemistry
Exchange energy
General chemistry
General chemistry
Functional groups ib chemistry
Organic vs inorganic chemistry
Class iv decay
Inspection auscultation percussion palpation
Chapter 4 posting to a general ledger
Body structure general survey
Normal pediatric vitals by age