Chemical Kinetics Rates and Mechanisms of Chemical Reactions
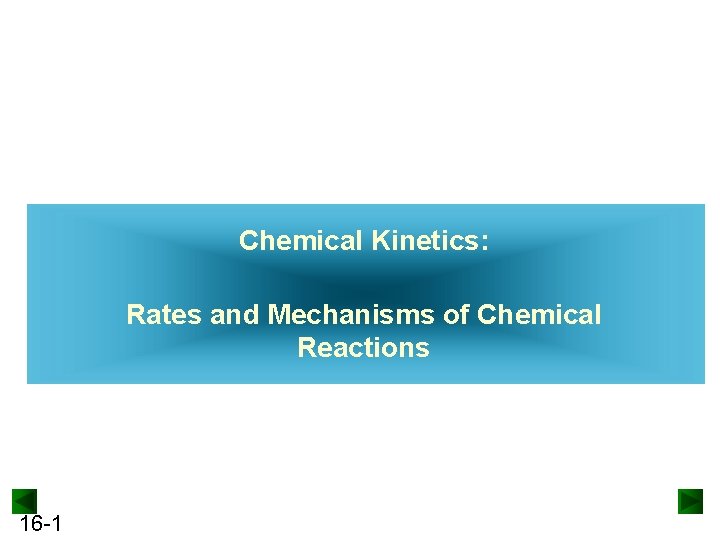
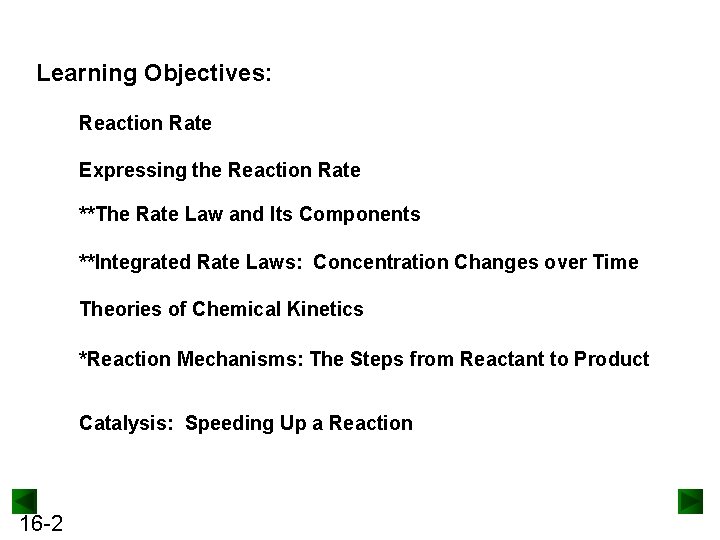
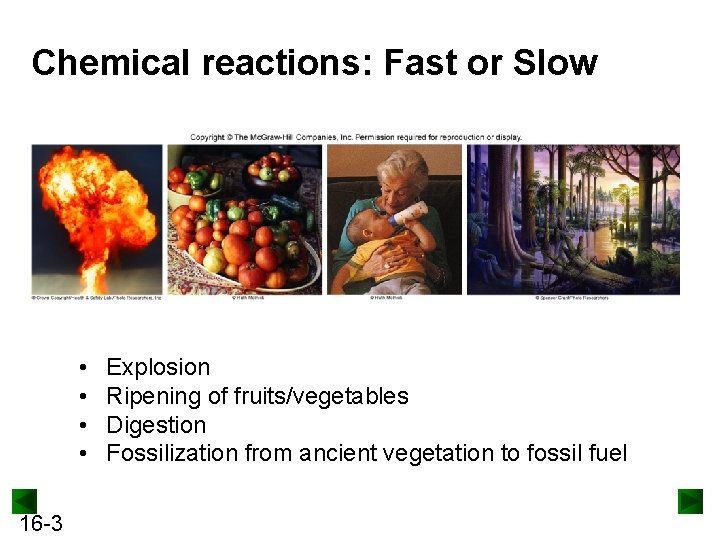
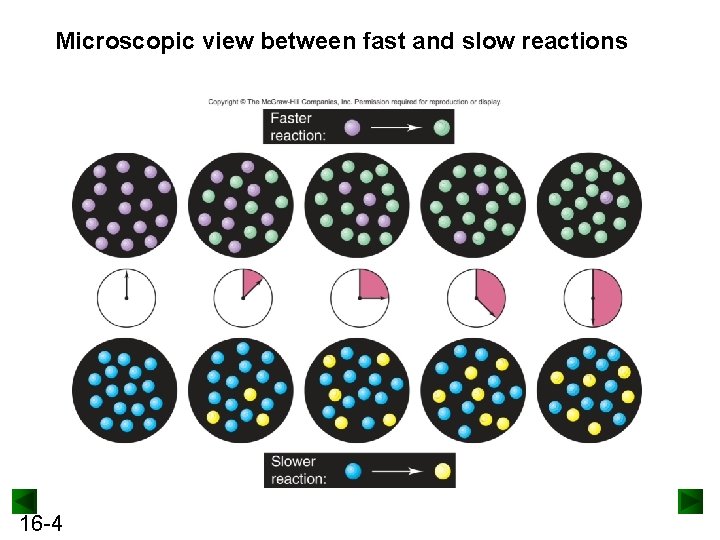
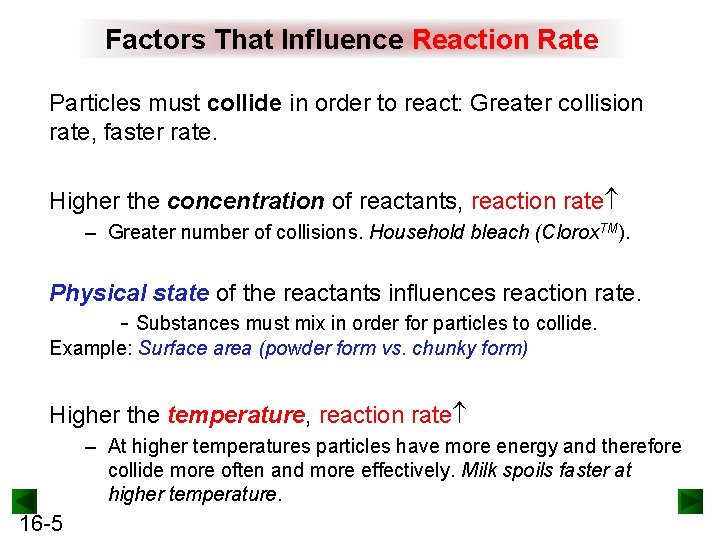
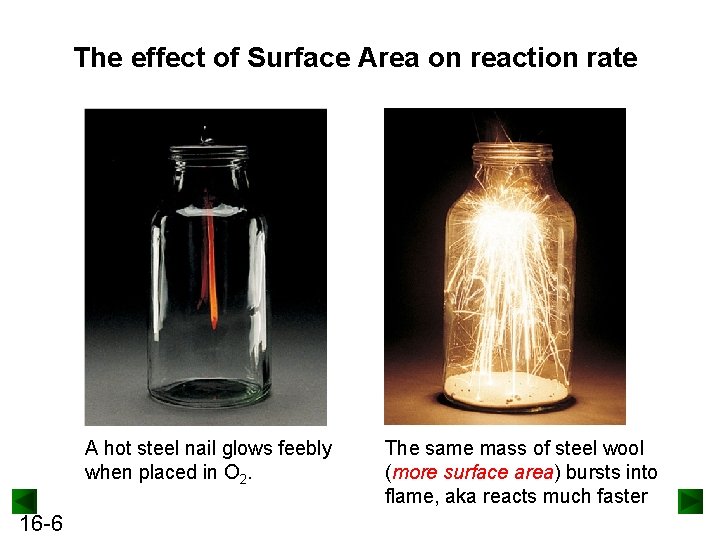
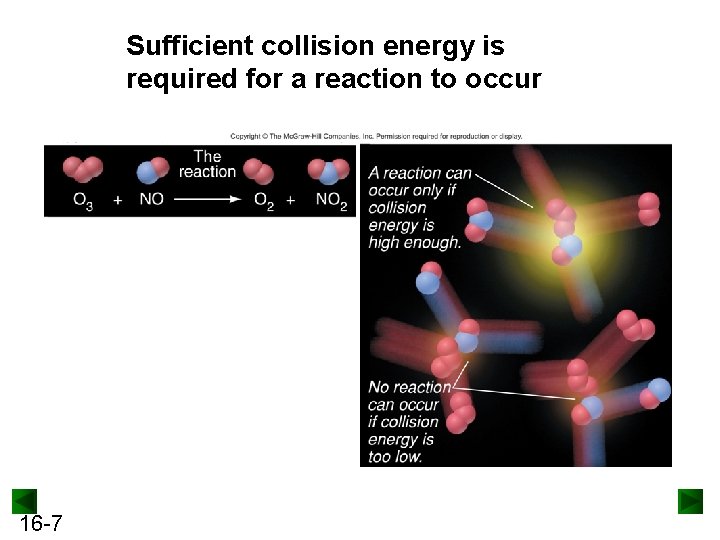
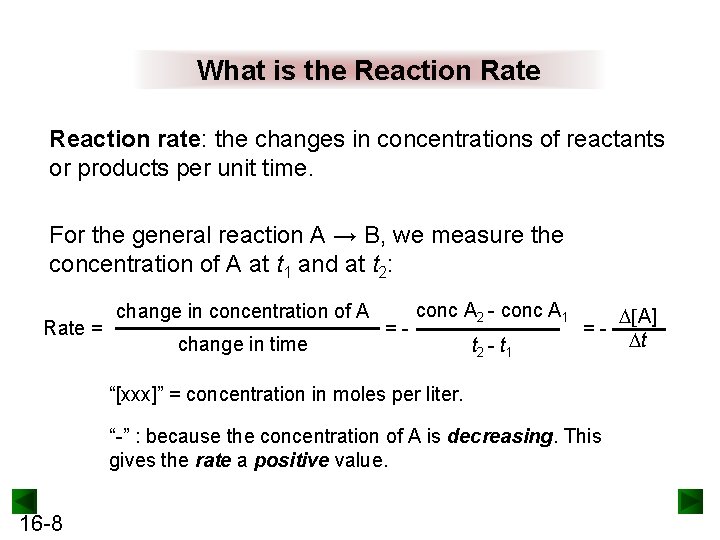
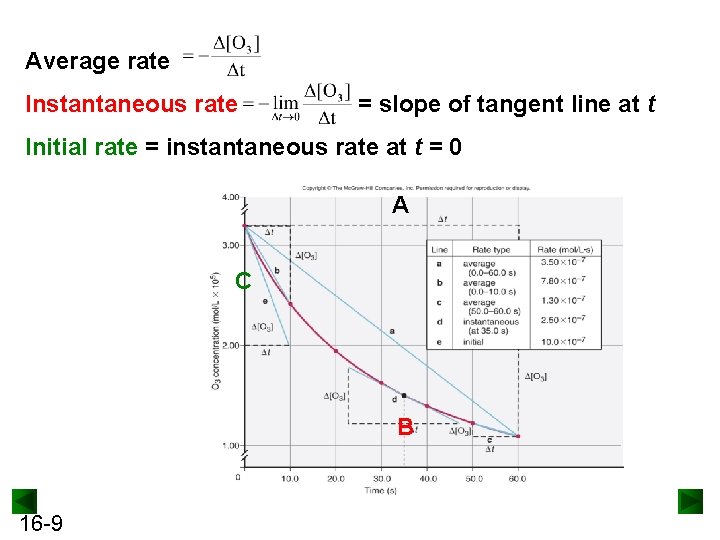
![Question: point A or B? ______ has the higher [O 3] ______ has higher Question: point A or B? ______ has the higher [O 3] ______ has higher](https://slidetodoc.com/presentation_image_h/0160eece20d4f5f28f0b0af4406d75c5/image-10.jpg)
![Plots of [reactant] and [product] vs. time. C 2 H 4 + O 3 Plots of [reactant] and [product] vs. time. C 2 H 4 + O 3](https://slidetodoc.com/presentation_image_h/0160eece20d4f5f28f0b0af4406d75c5/image-11.jpg)
![Plots of [reactant] and [product] vs. time. H 2 + I 2 → 2 Plots of [reactant] and [product] vs. time. H 2 + I 2 → 2](https://slidetodoc.com/presentation_image_h/0160eece20d4f5f28f0b0af4406d75c5/image-12.jpg)
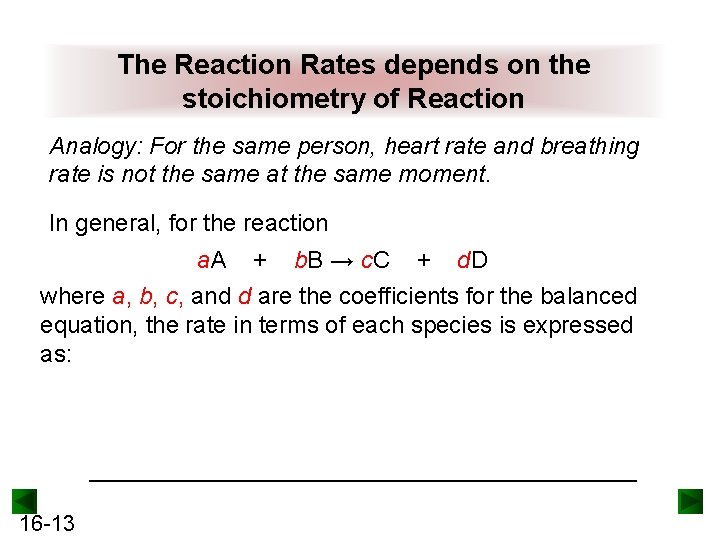
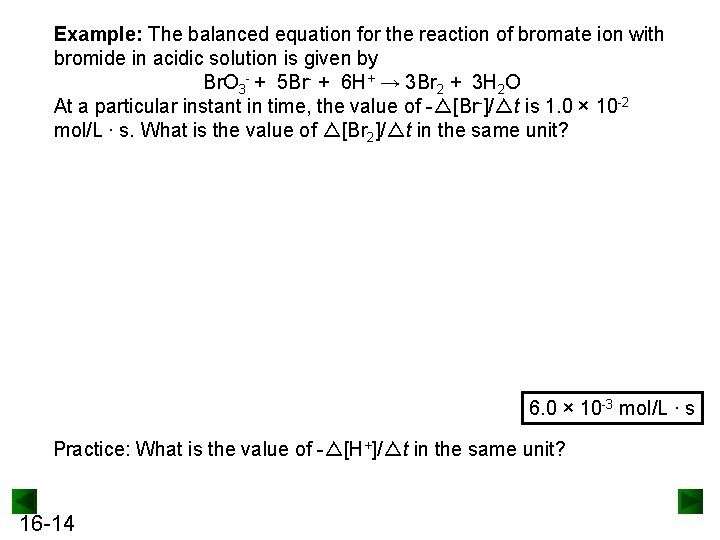
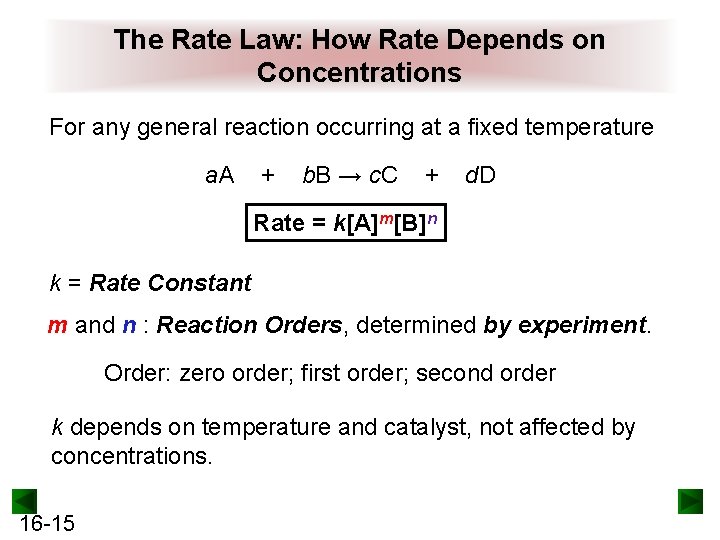
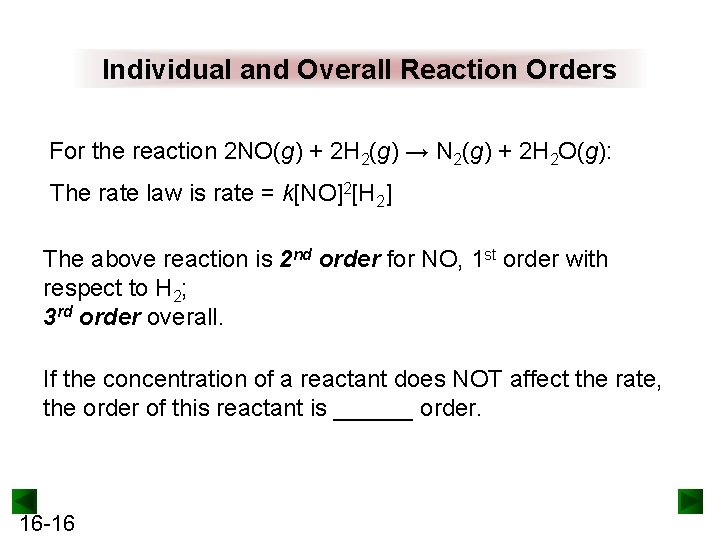
![Plots of Reactant concentration, [A], vs. Time 16 -17 Plots of Reactant concentration, [A], vs. Time 16 -17](https://slidetodoc.com/presentation_image_h/0160eece20d4f5f28f0b0af4406d75c5/image-17.jpg)
![Plots of Rate vs. Reactant Concentration [A] 16 -18 Plots of Rate vs. Reactant Concentration [A] 16 -18](https://slidetodoc.com/presentation_image_h/0160eece20d4f5f28f0b0af4406d75c5/image-18.jpg)
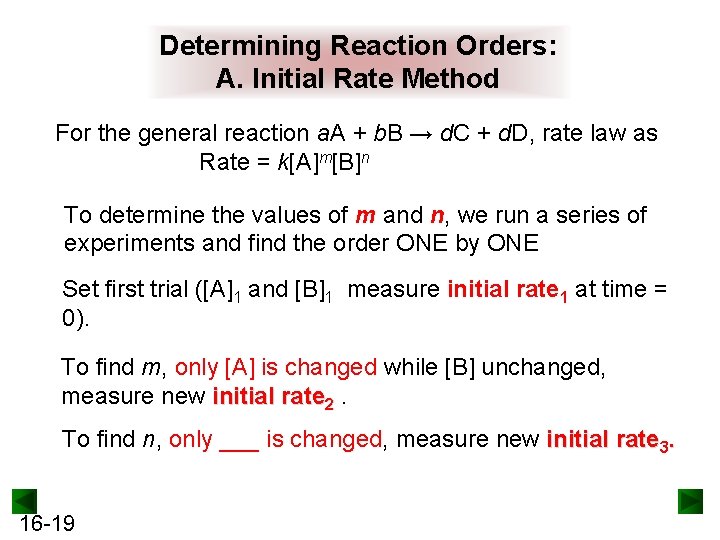
![Initial Rate Method to Determine the order Experiment Initial Rate (mol/L·s) Initial [A] (mol/L) Initial Rate Method to Determine the order Experiment Initial Rate (mol/L·s) Initial [A] (mol/L)](https://slidetodoc.com/presentation_image_h/0160eece20d4f5f28f0b0af4406d75c5/image-20.jpg)
![Finding the order with respect to A Rate = k[A]m[B]n Trial Initial Rate (mol/L·s) Finding the order with respect to A Rate = k[A]m[B]n Trial Initial Rate (mol/L·s)](https://slidetodoc.com/presentation_image_h/0160eece20d4f5f28f0b0af4406d75c5/image-21.jpg)
![Practice: Find the order with respect to B Rate = k[A]m[B]n Trial Initial Rate Practice: Find the order with respect to B Rate = k[A]m[B]n Trial Initial Rate](https://slidetodoc.com/presentation_image_h/0160eece20d4f5f28f0b0af4406d75c5/image-22.jpg)
![Practice: Finding the rate constant k Initial Rate = Trial (mol/L·s) Initial [A] (mol/L) Practice: Finding the rate constant k Initial Rate = Trial (mol/L·s) Initial [A] (mol/L)](https://slidetodoc.com/presentation_image_h/0160eece20d4f5f28f0b0af4406d75c5/image-23.jpg)
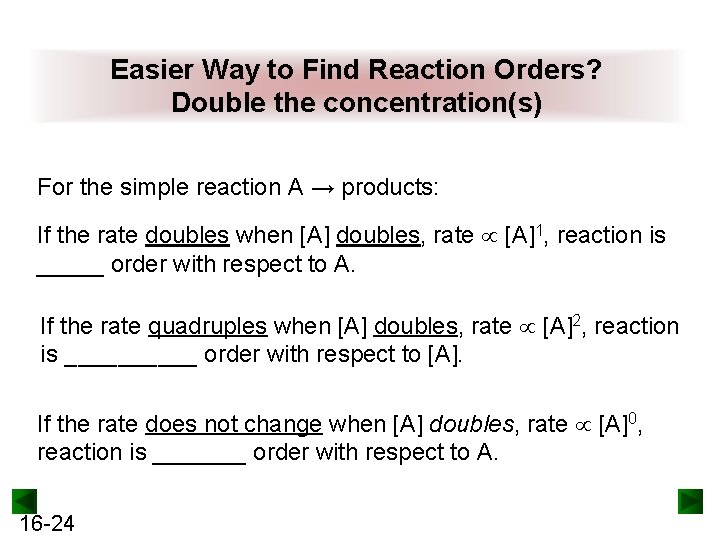
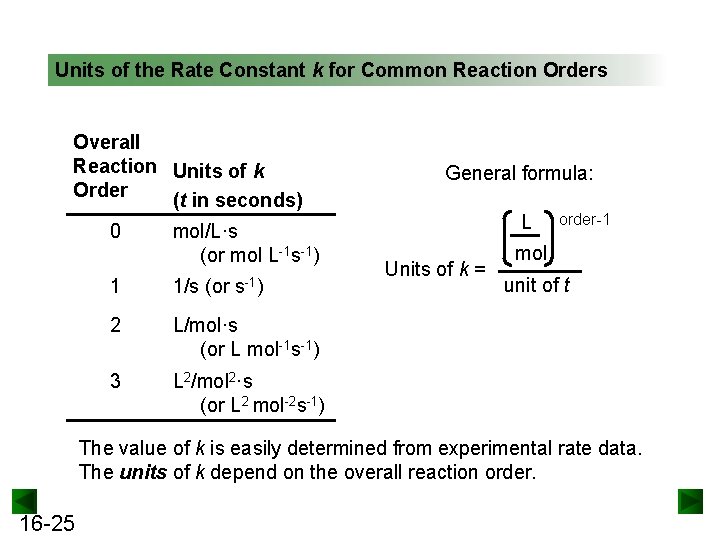
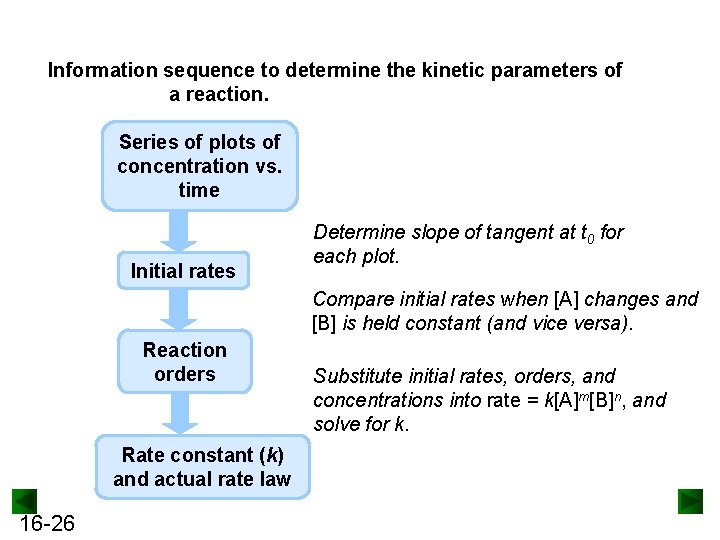
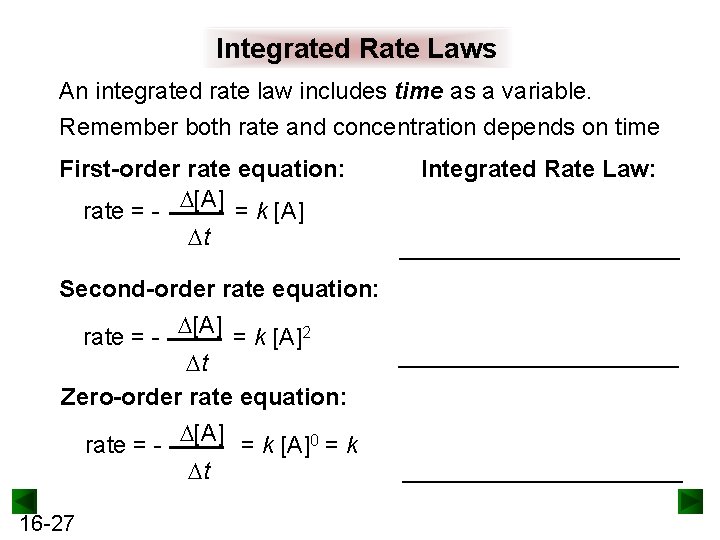
![Graphical presentation of 1 st order reaction First-order reaction integrated rate law [A]0 = Graphical presentation of 1 st order reaction First-order reaction integrated rate law [A]0 =](https://slidetodoc.com/presentation_image_h/0160eece20d4f5f28f0b0af4406d75c5/image-28.jpg)
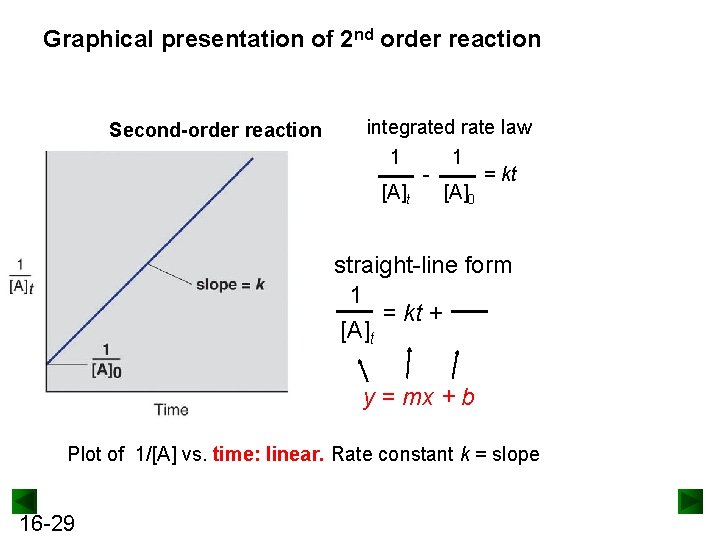
![Graphical presentation of zero order reaction Zero-order reaction integrated rate law [A]t - [A]0 Graphical presentation of zero order reaction Zero-order reaction integrated rate law [A]t - [A]0](https://slidetodoc.com/presentation_image_h/0160eece20d4f5f28f0b0af4406d75c5/image-30.jpg)
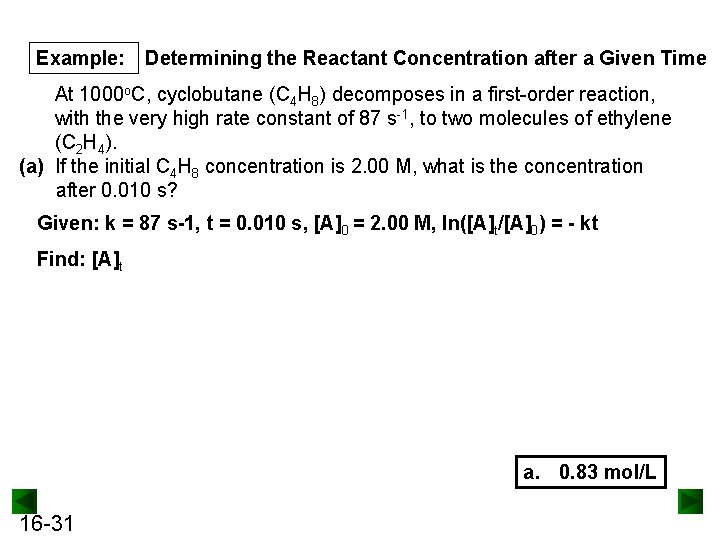
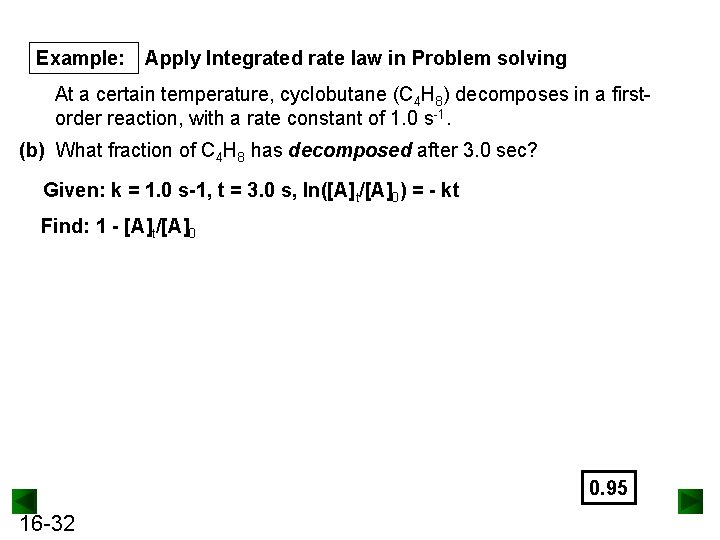
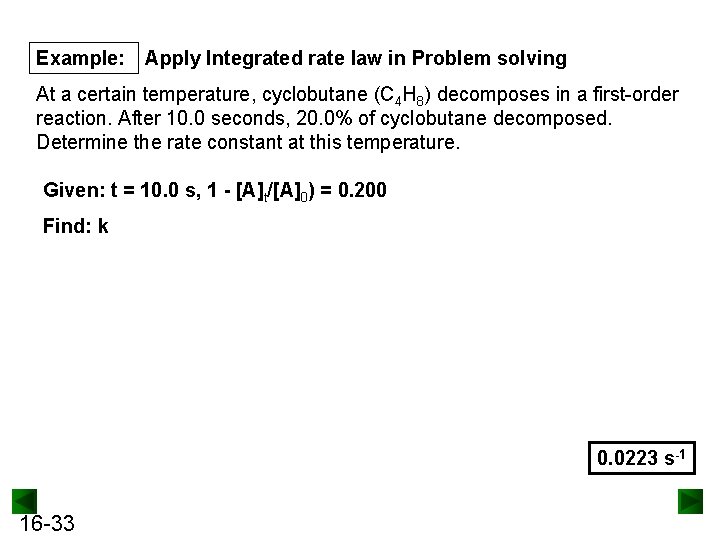
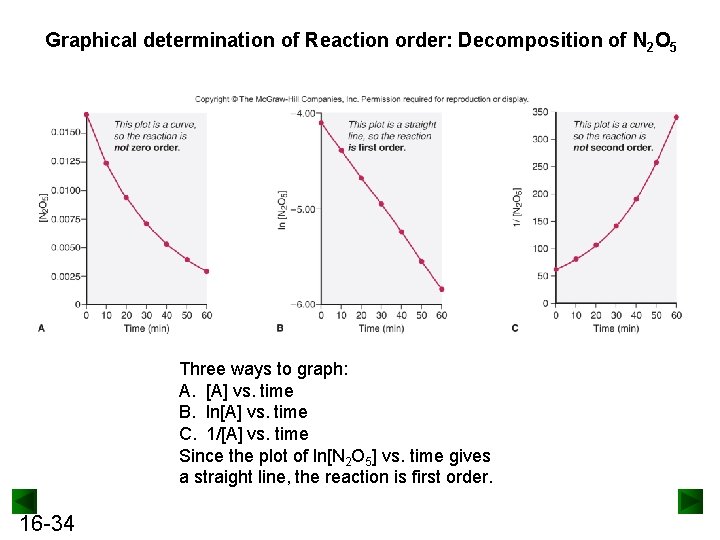
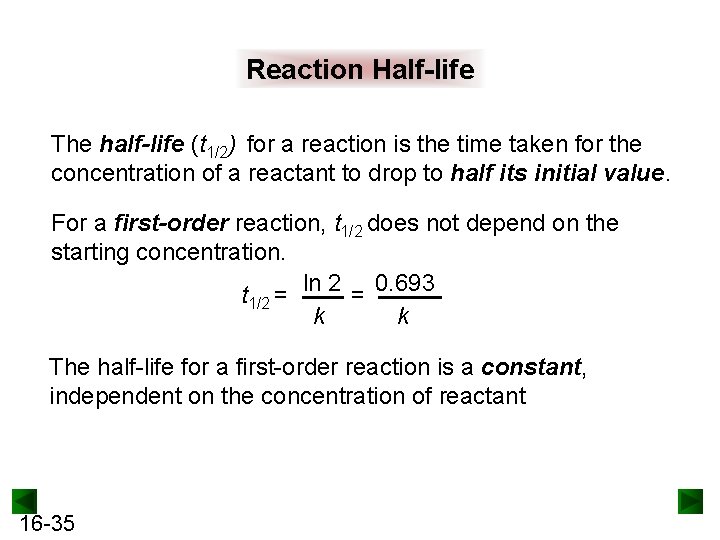
![[N 2 O 5] vs. time for three reaction half-lives. for a first-order process [N 2 O 5] vs. time for three reaction half-lives. for a first-order process](https://slidetodoc.com/presentation_image_h/0160eece20d4f5f28f0b0af4406d75c5/image-36.jpg)
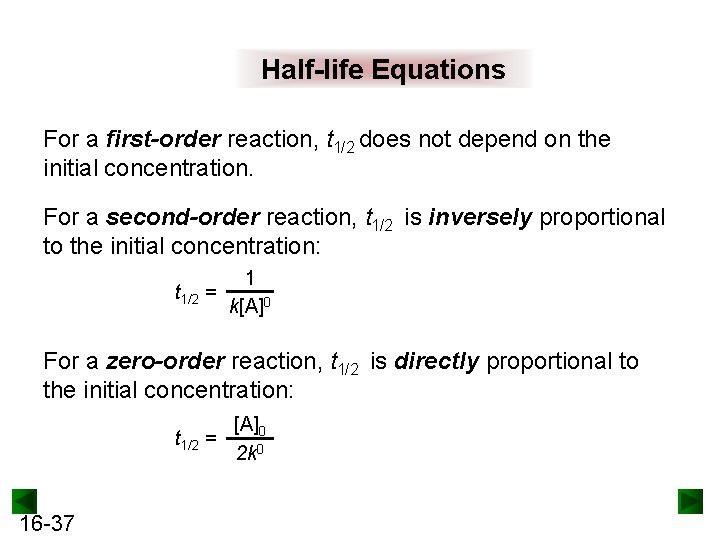
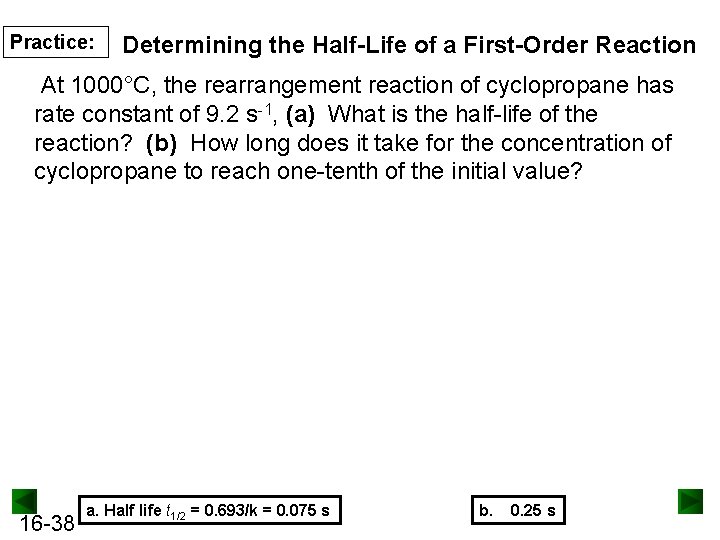
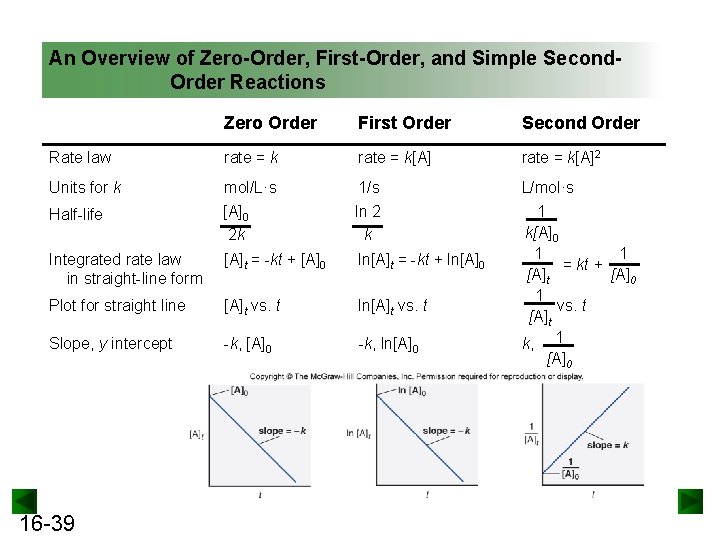
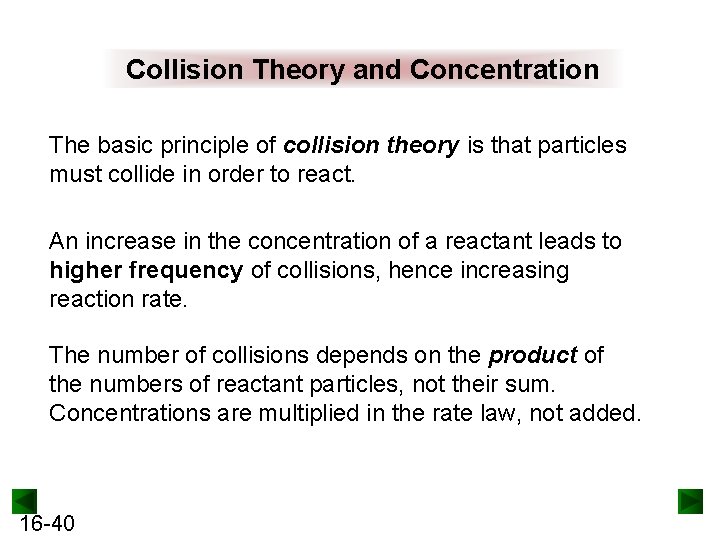
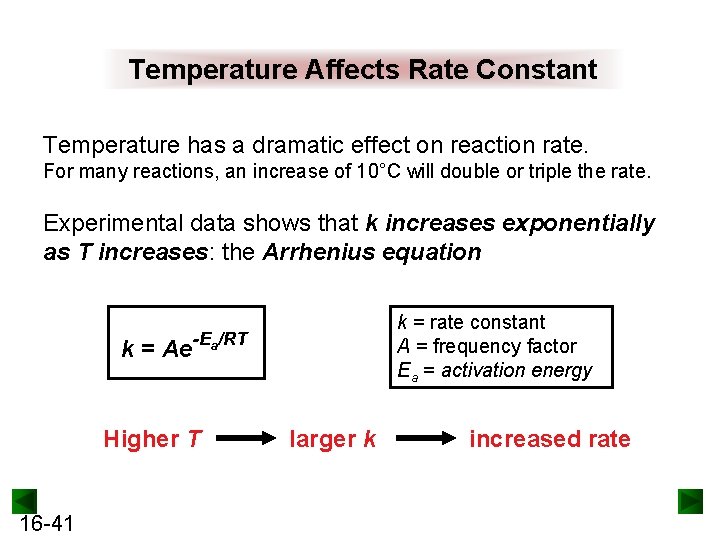
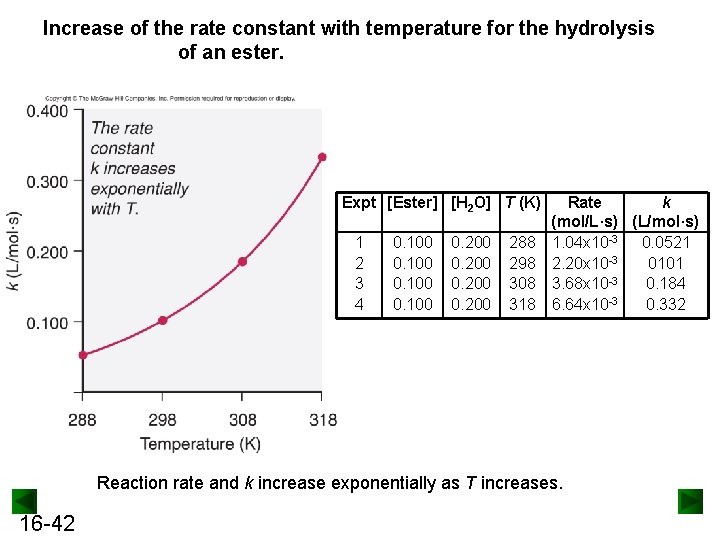
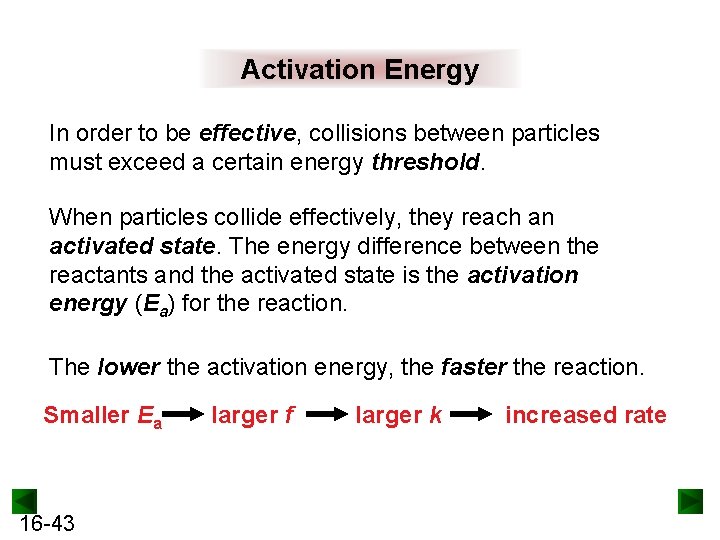
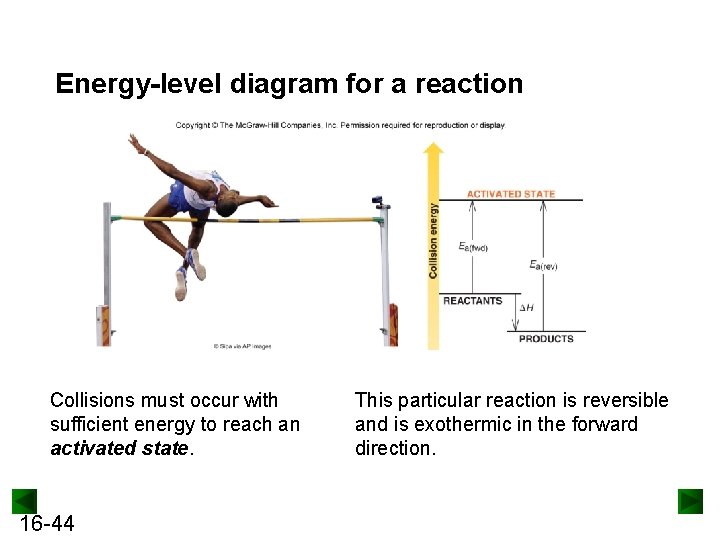
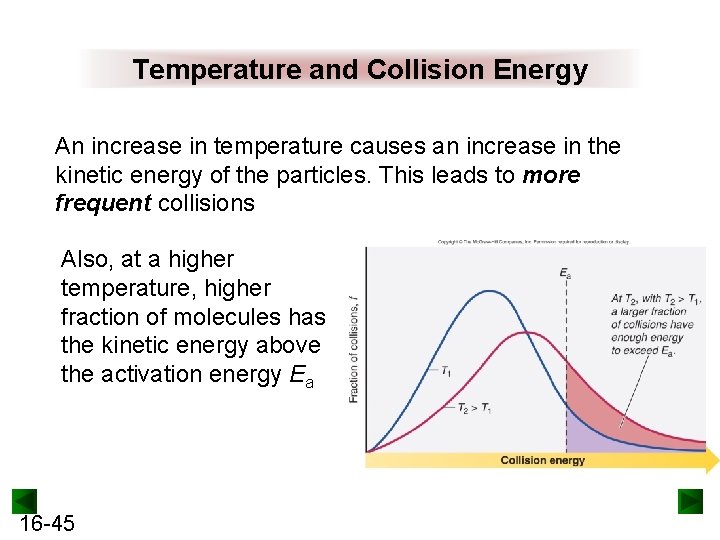
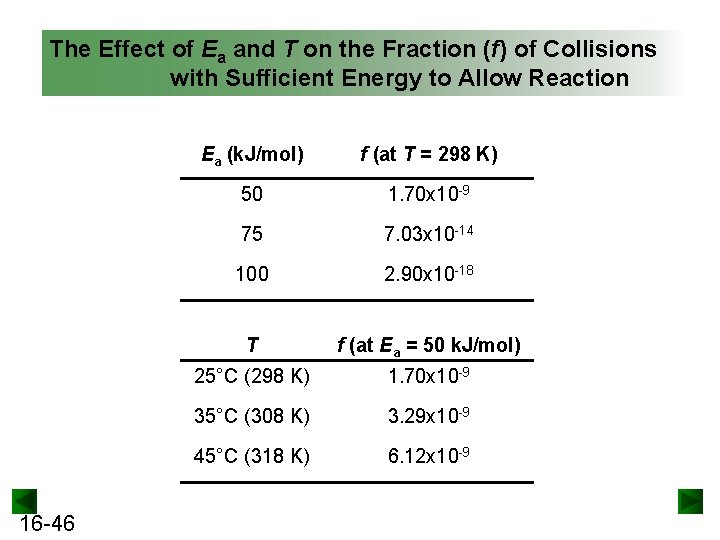
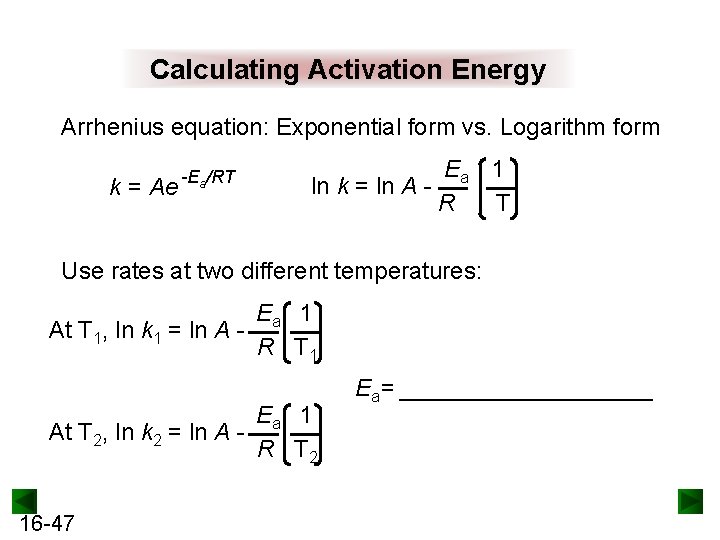
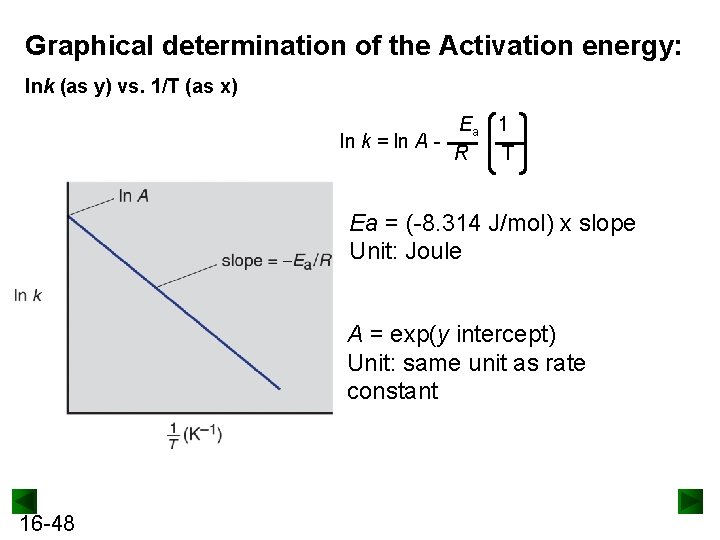
![Find Activation Energy and A factor from graph Expt [Ester] [H 2 O] T Find Activation Energy and A factor from graph Expt [Ester] [H 2 O] T](https://slidetodoc.com/presentation_image_h/0160eece20d4f5f28f0b0af4406d75c5/image-49.jpg)
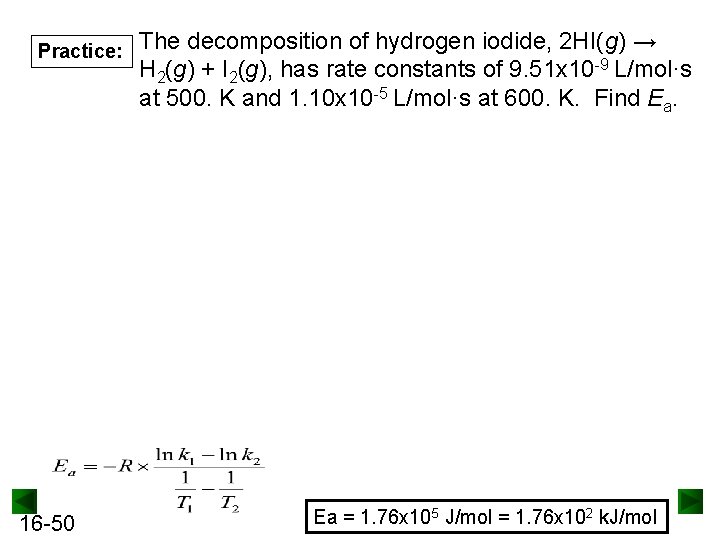
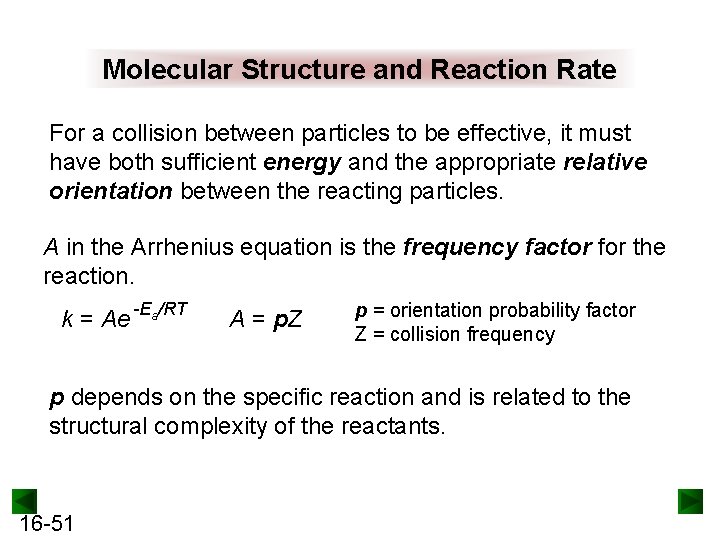
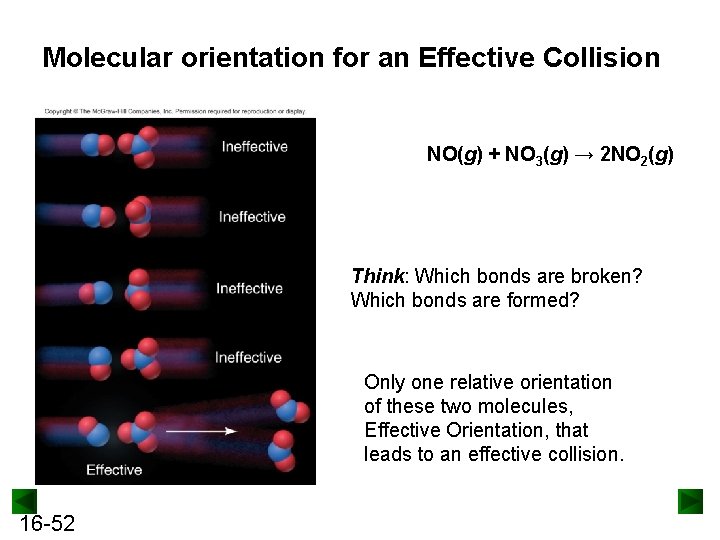
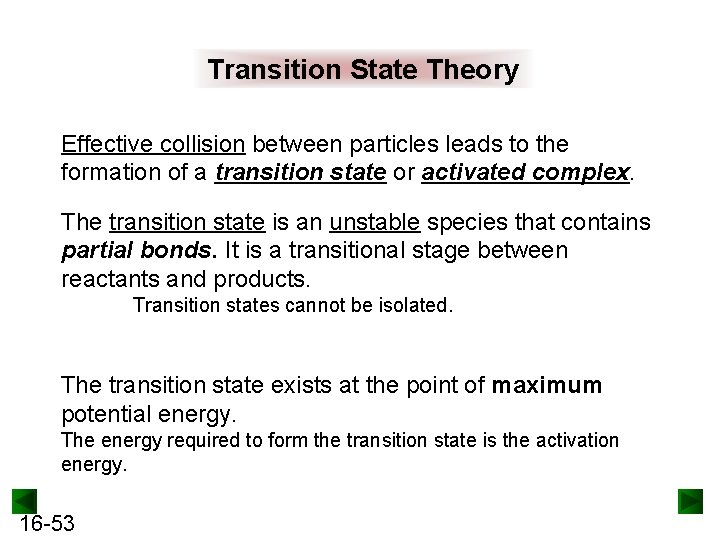
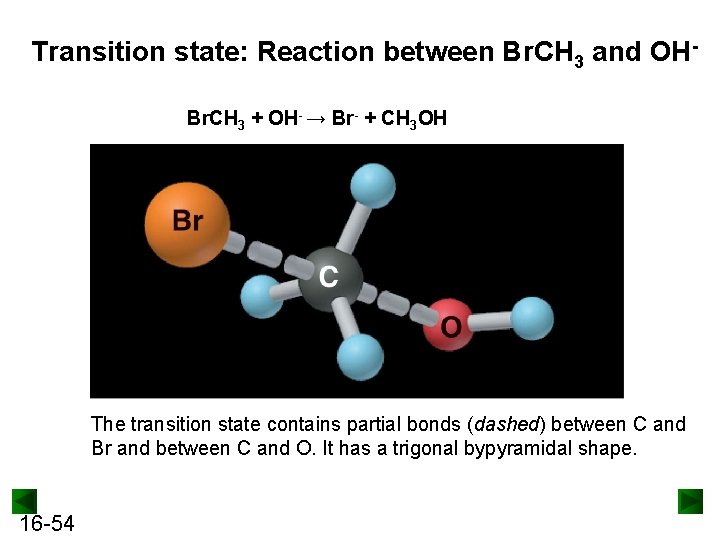
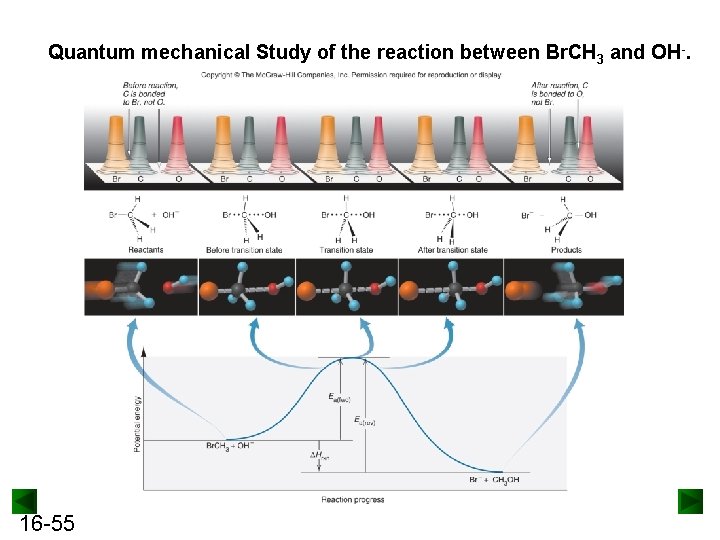
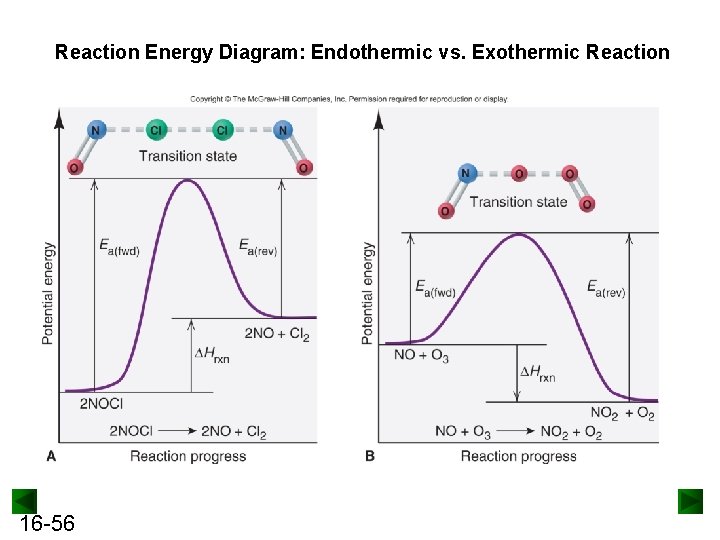
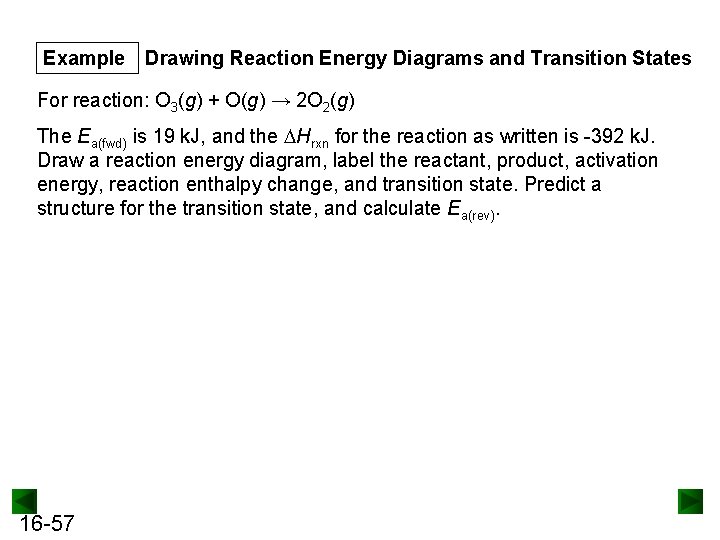
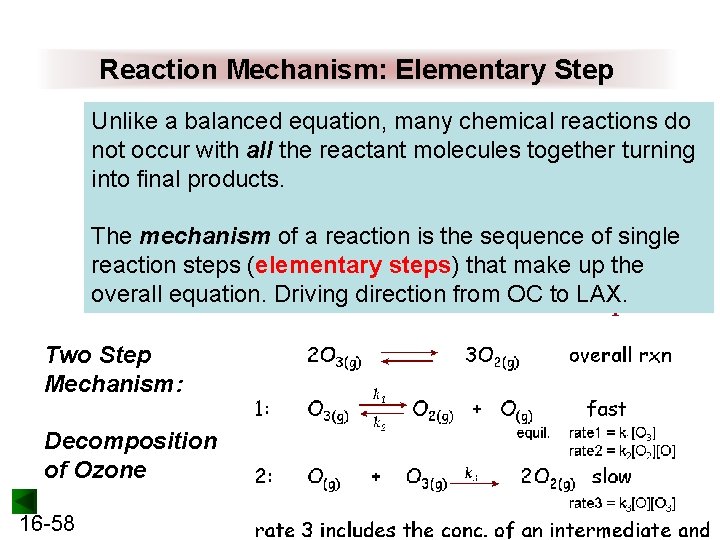
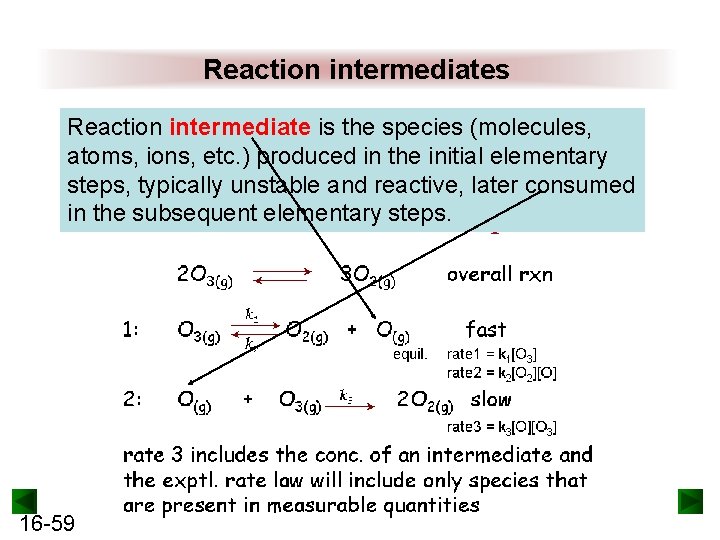
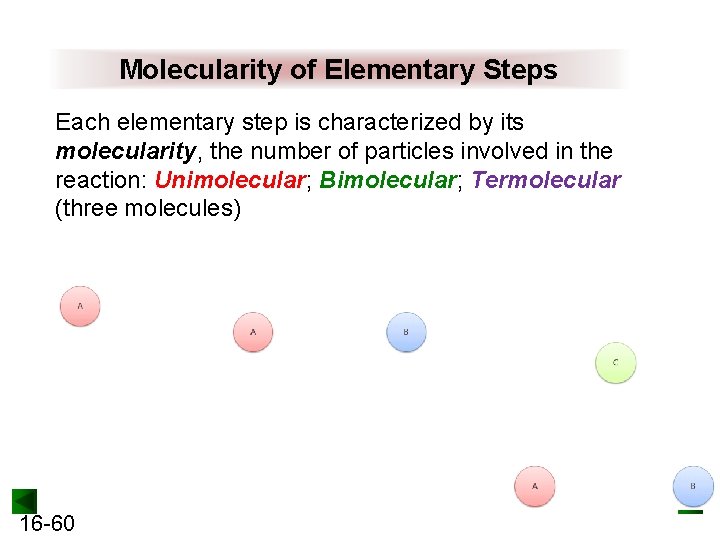
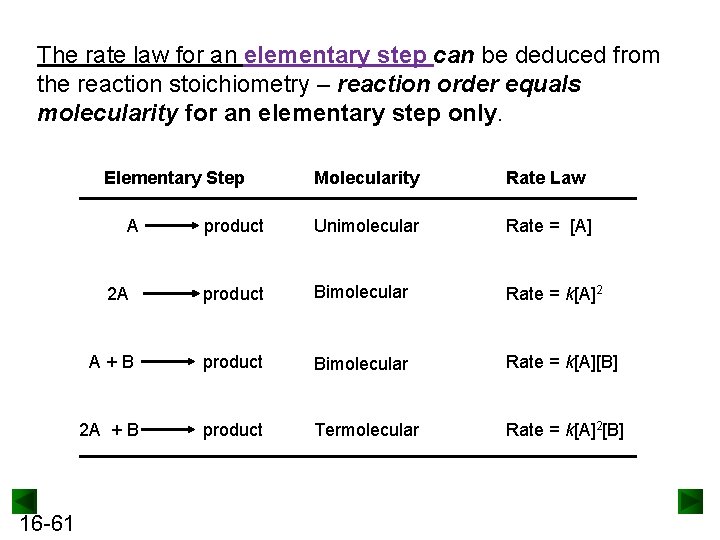
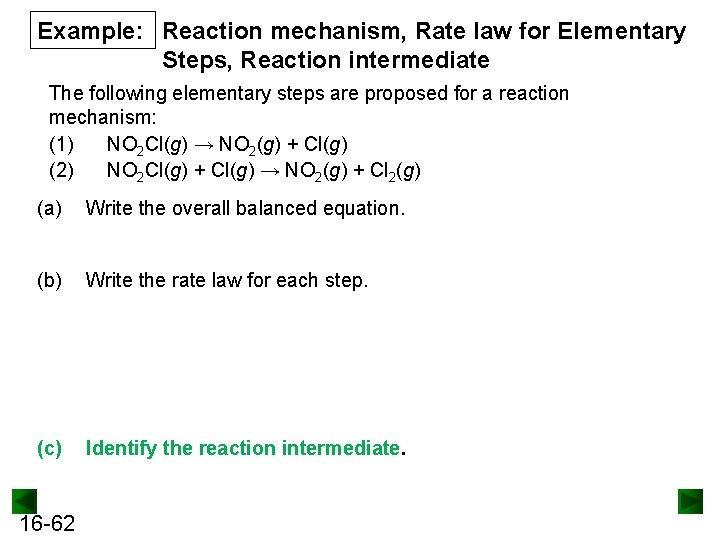
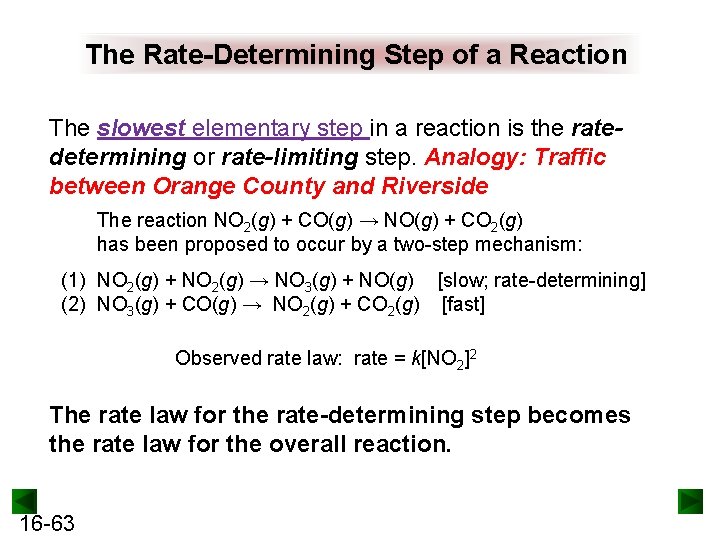
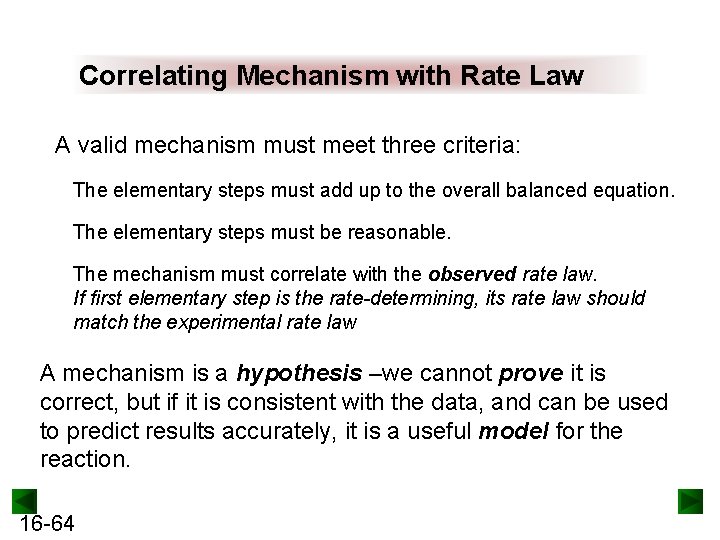
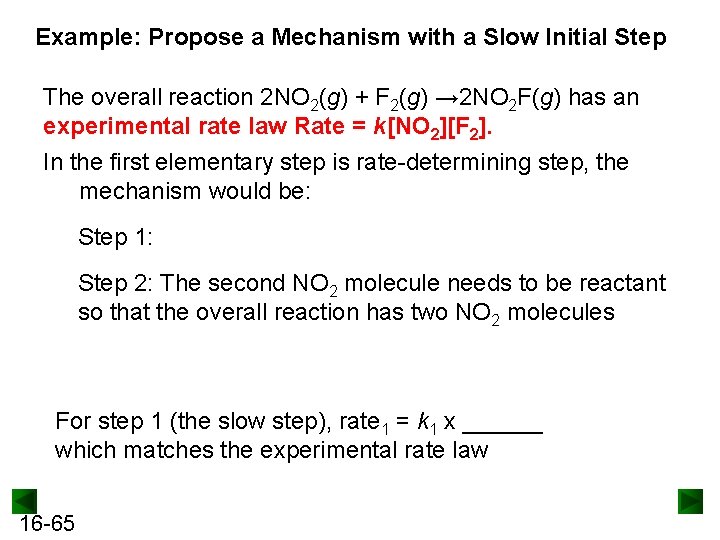
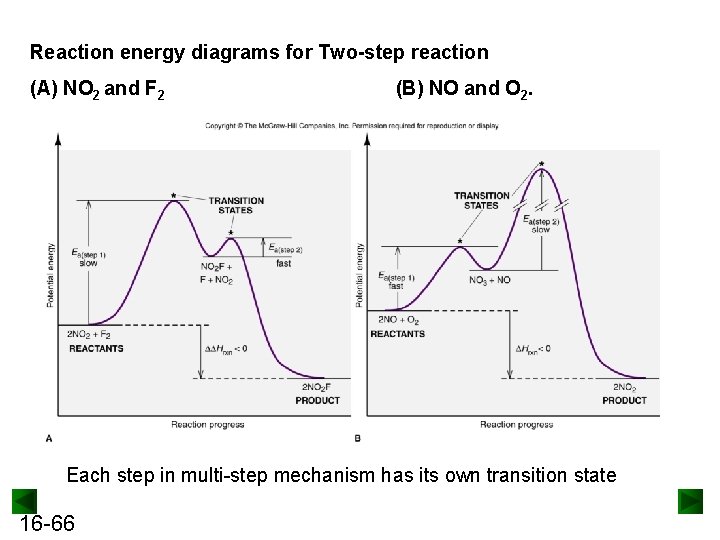
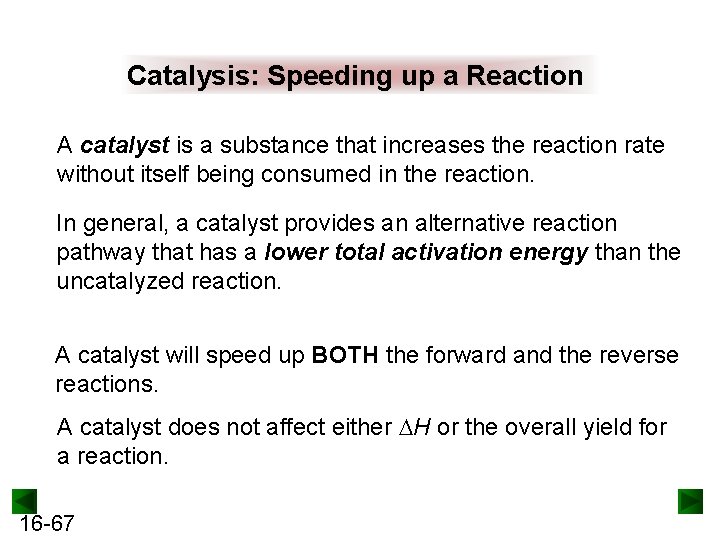
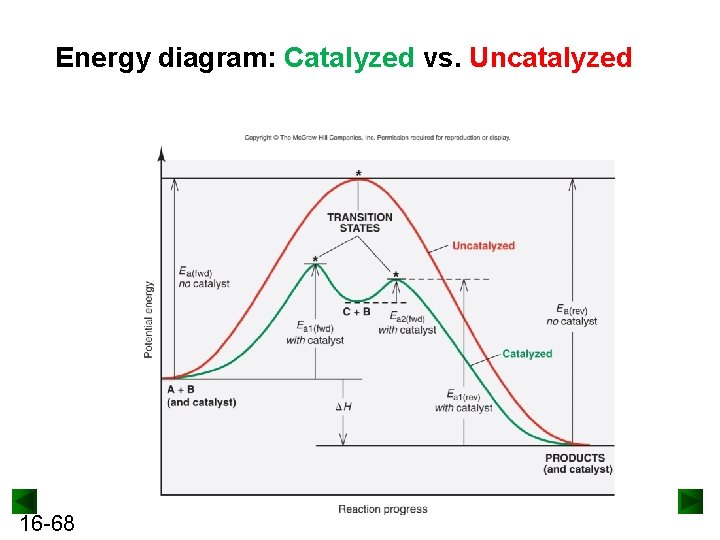
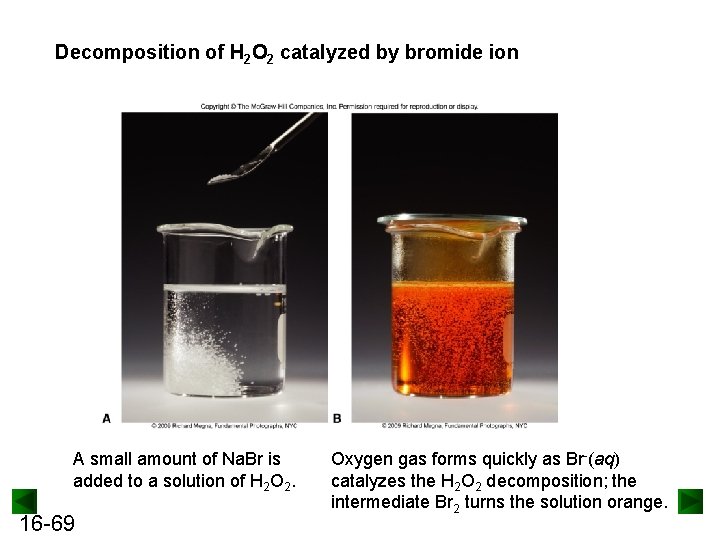
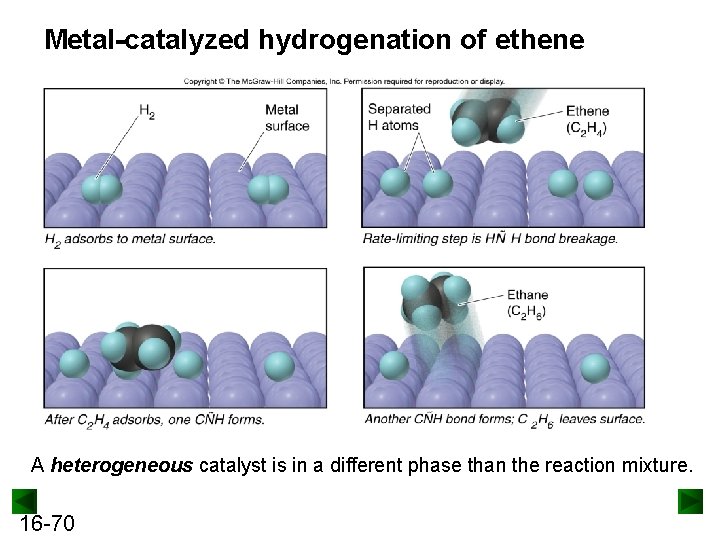
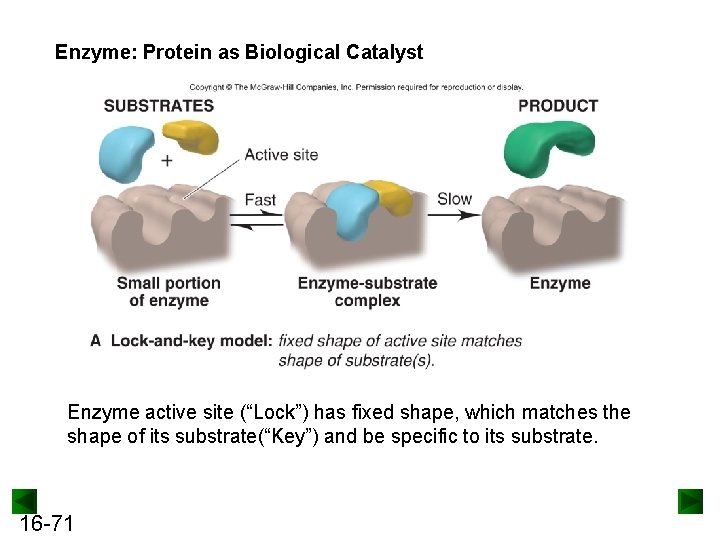
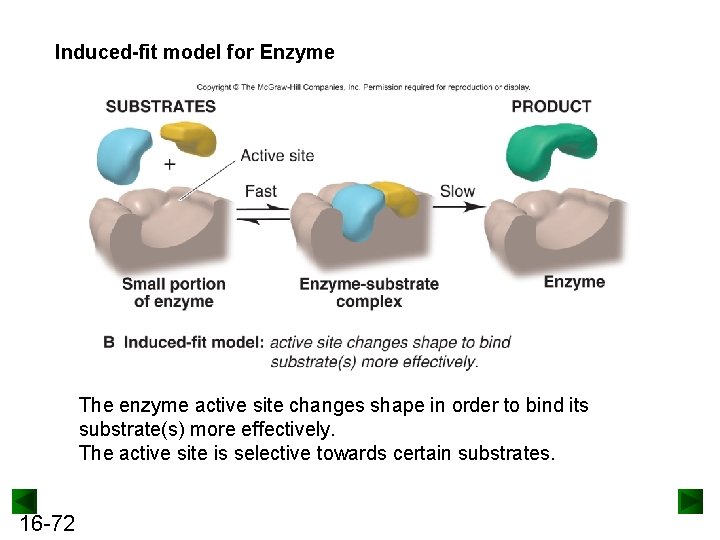
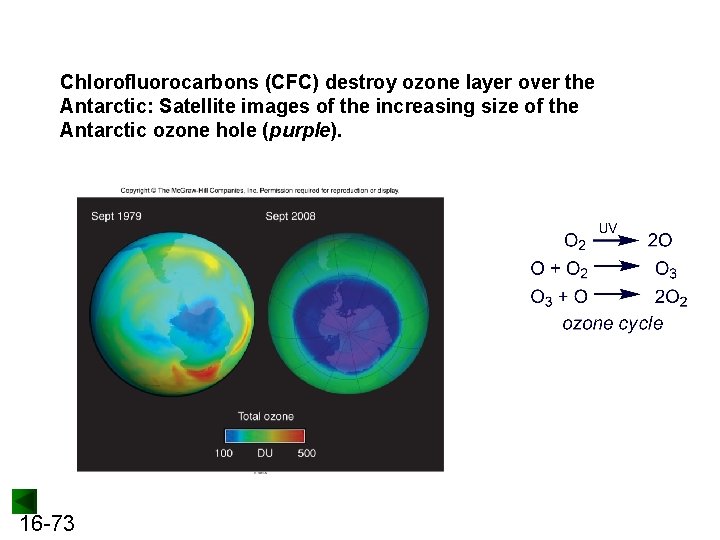
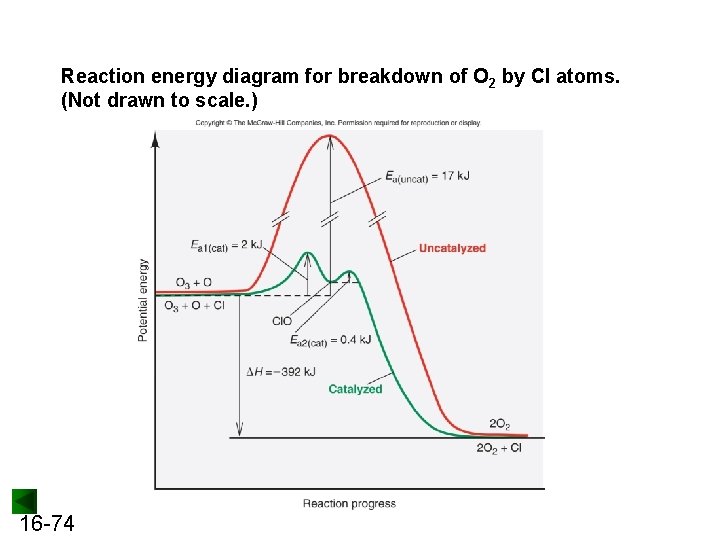
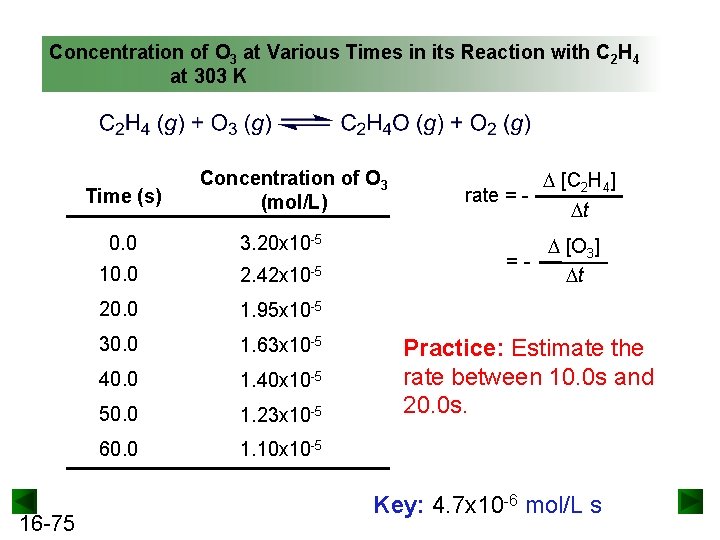
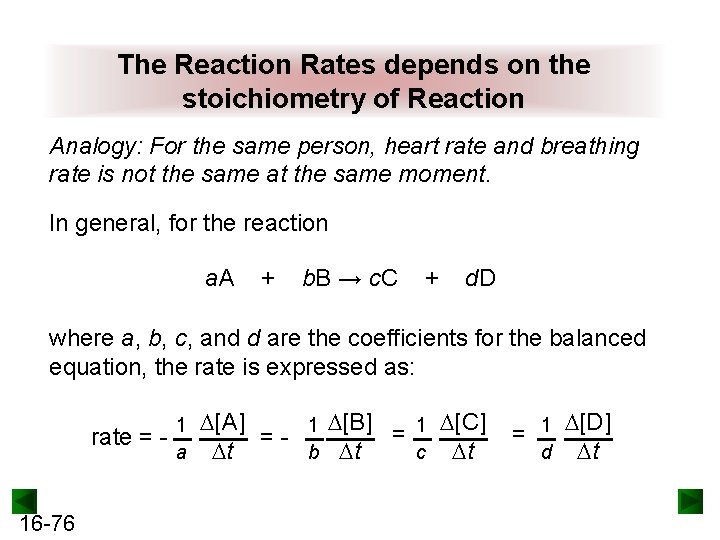
![Rate = k[A]m[B]n Finding m, the order with respect to A: We compare experiments Rate = k[A]m[B]n Finding m, the order with respect to A: We compare experiments](https://slidetodoc.com/presentation_image_h/0160eece20d4f5f28f0b0af4406d75c5/image-77.jpg)
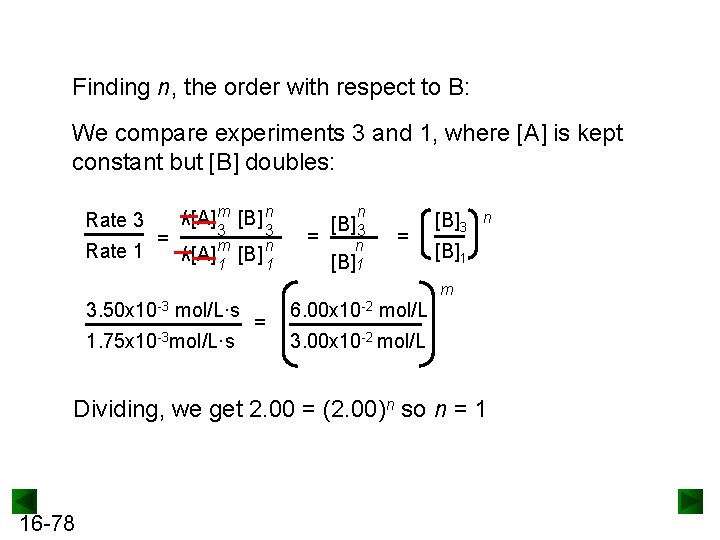
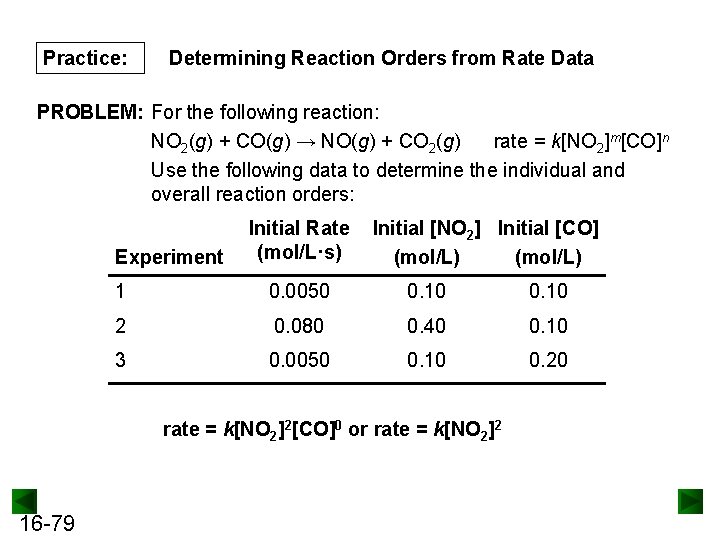
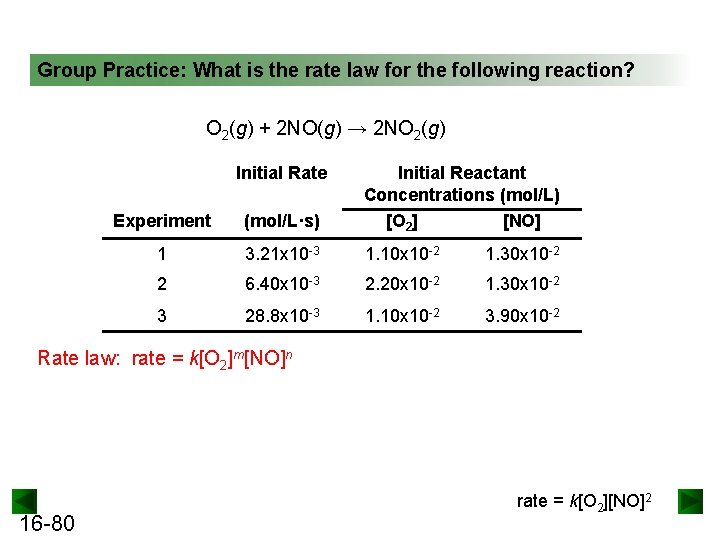
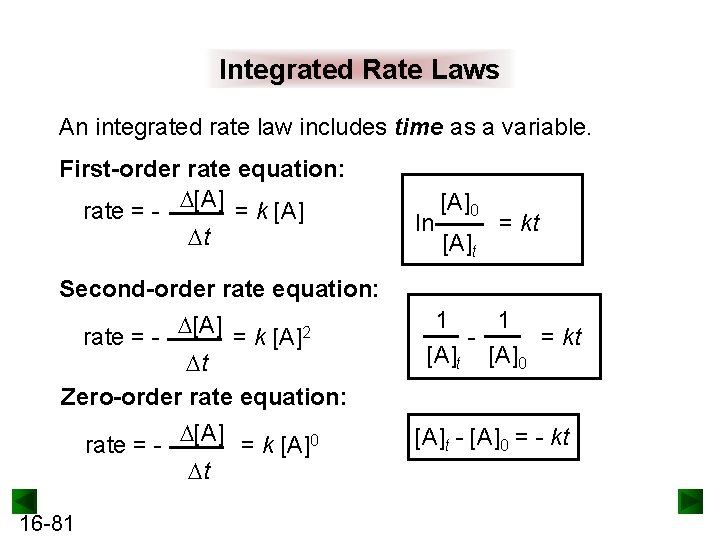
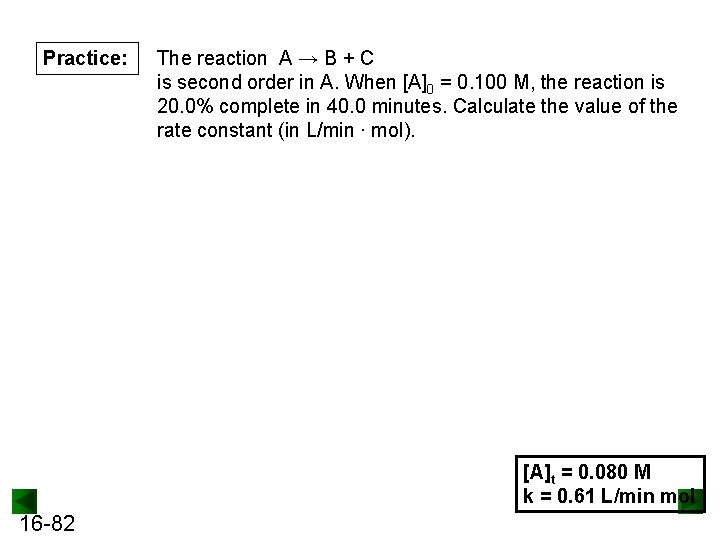
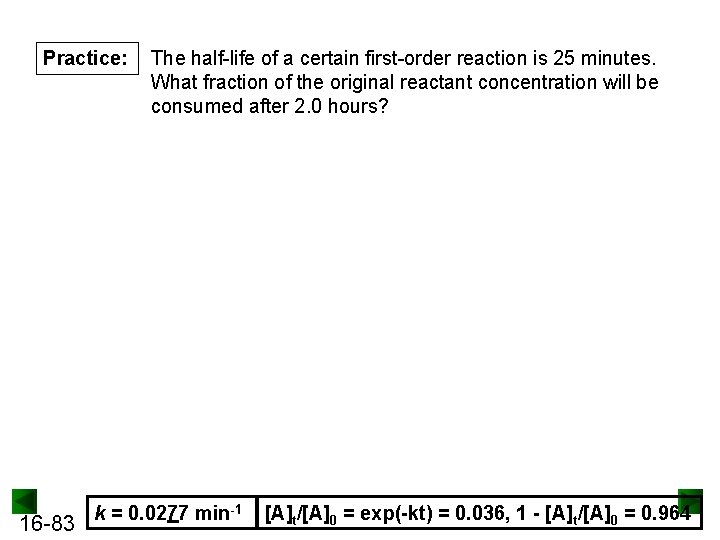
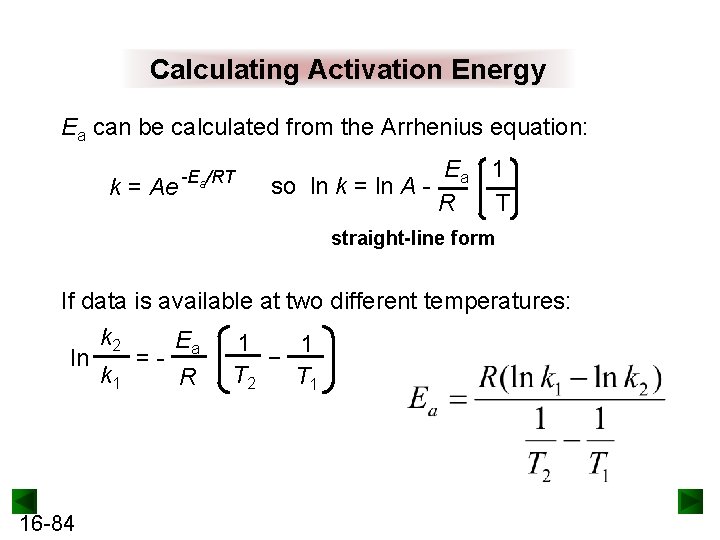
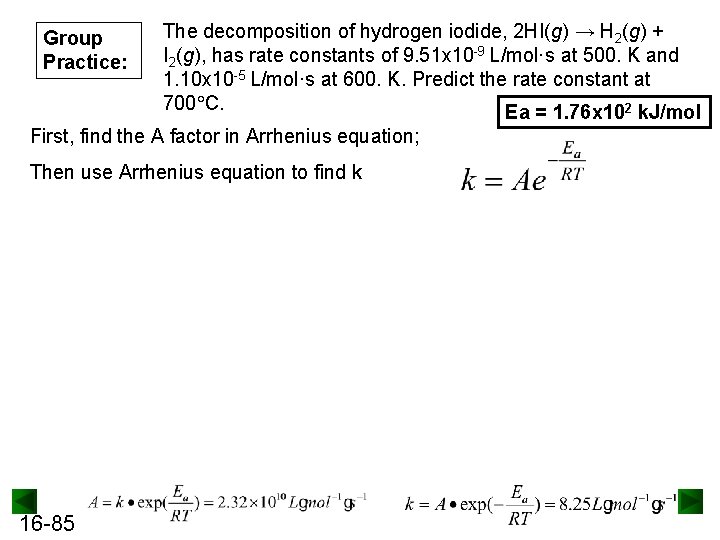
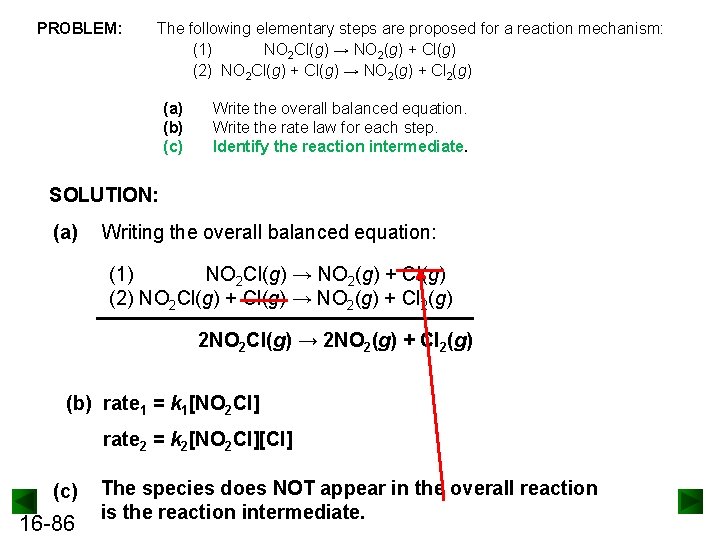
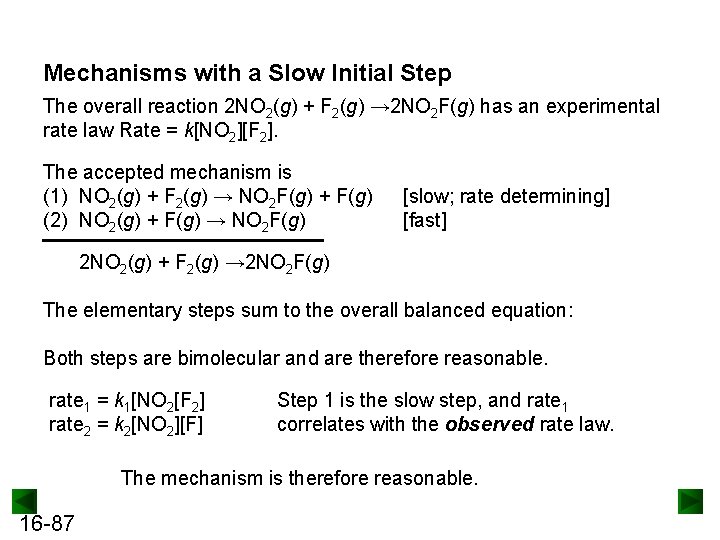
- Slides: 87
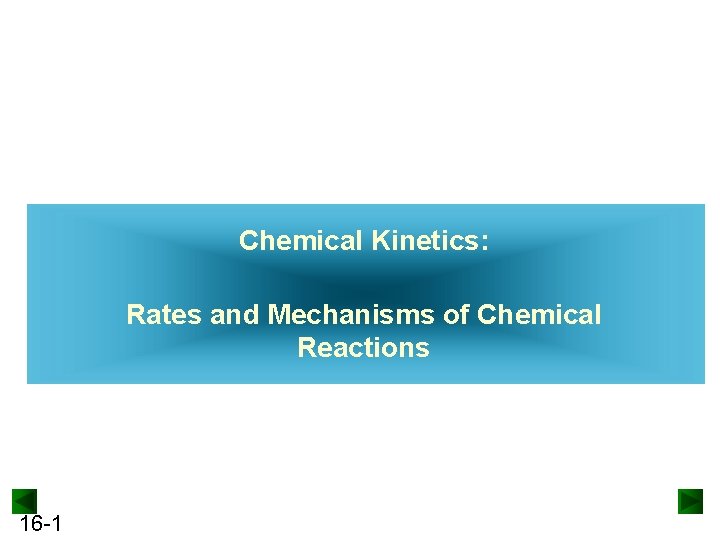
Chemical Kinetics: Rates and Mechanisms of Chemical Reactions 16 -1
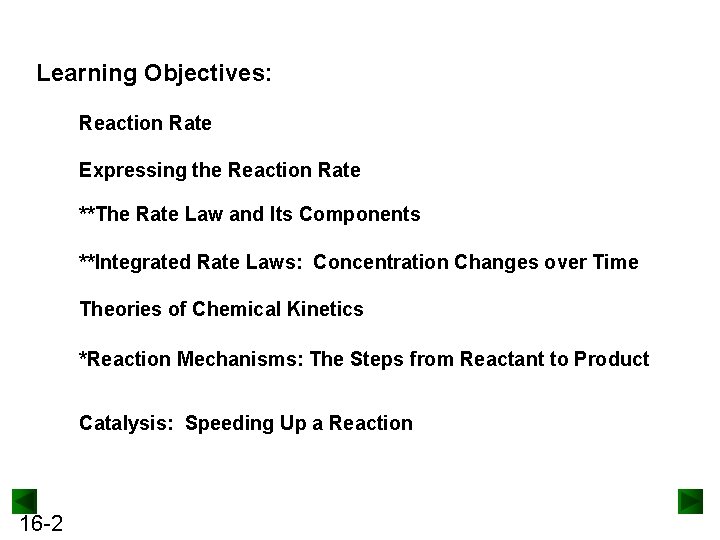
Learning Objectives: Reaction Rate Expressing the Reaction Rate **The Rate Law and Its Components **Integrated Rate Laws: Concentration Changes over Time Theories of Chemical Kinetics *Reaction Mechanisms: The Steps from Reactant to Product Catalysis: Speeding Up a Reaction 16 -2
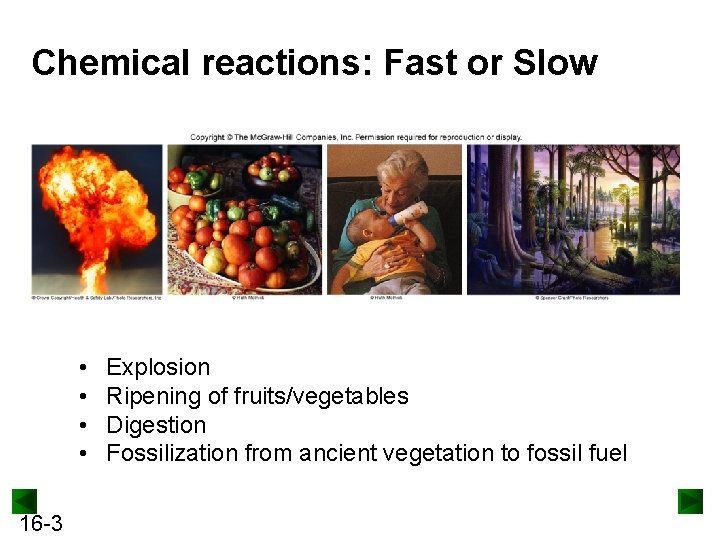
Chemical reactions: Fast or Slow • • 16 -3 Explosion Ripening of fruits/vegetables Digestion Fossilization from ancient vegetation to fossil fuel
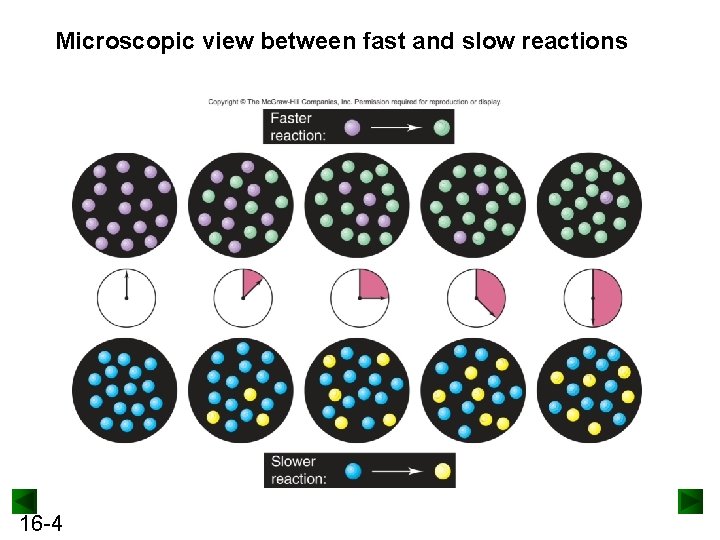
Microscopic view between fast and slow reactions 16 -4
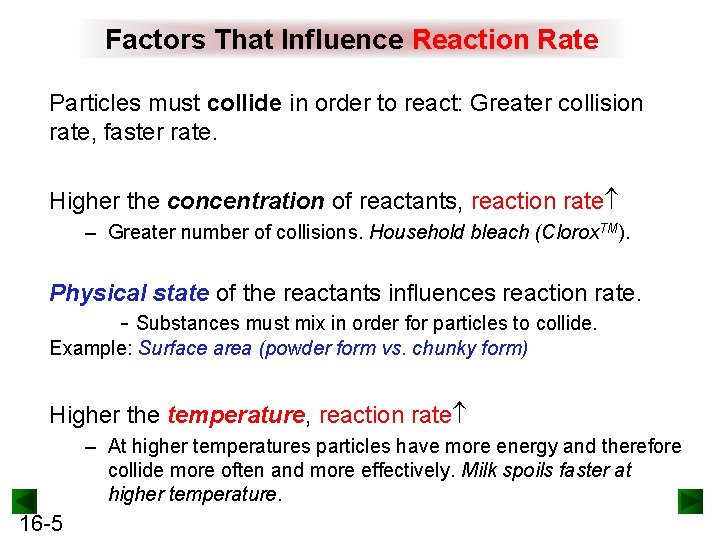
Factors That Influence Reaction Rate Particles must collide in order to react: Greater collision rate, faster rate. Higher the concentration of reactants, reaction rate – Greater number of collisions. Household bleach (Clorox. TM). Physical state of the reactants influences reaction rate. - Substances must mix in order for particles to collide. Example: Surface area (powder form vs. chunky form) Higher the temperature, reaction rate – At higher temperatures particles have more energy and therefore collide more often and more effectively. Milk spoils faster at higher temperature. 16 -5
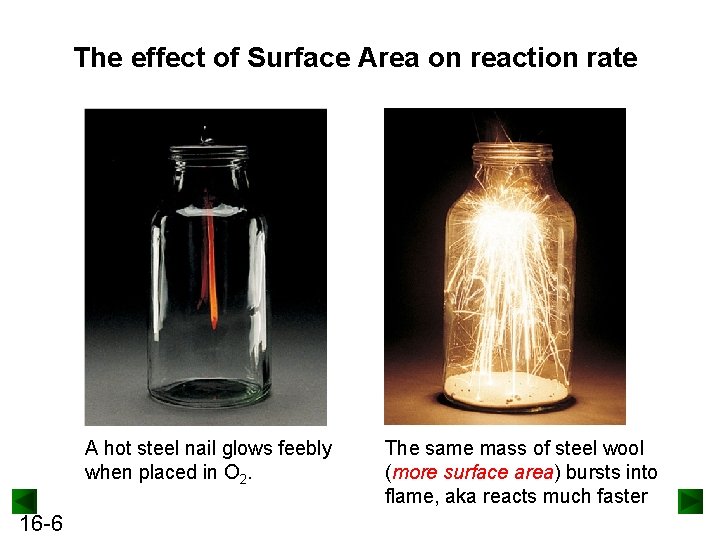
The effect of Surface Area on reaction rate A hot steel nail glows feebly when placed in O 2. 16 -6 The same mass of steel wool (more surface area) bursts into flame, aka reacts much faster
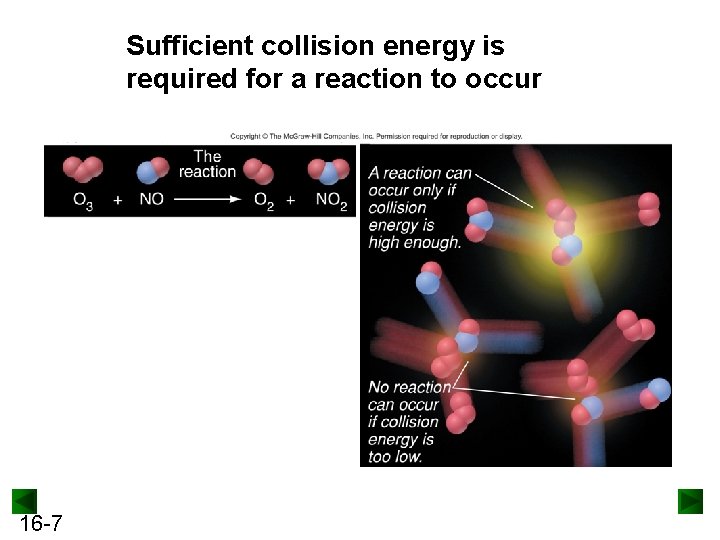
Sufficient collision energy is required for a reaction to occur 16 -7
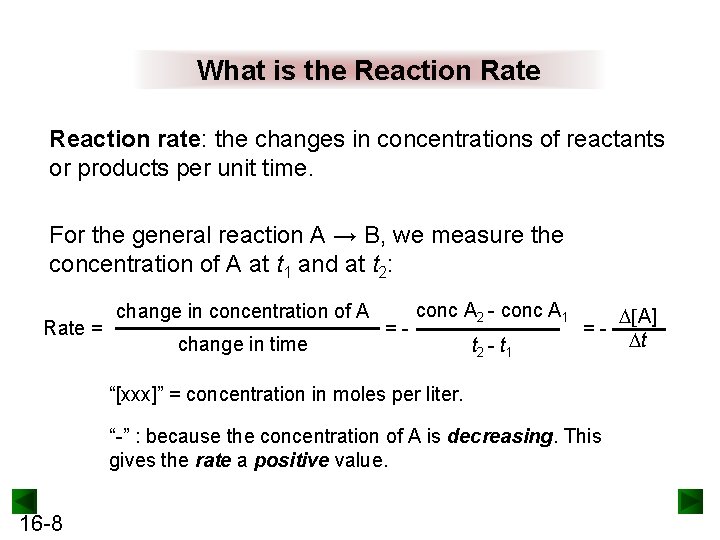
What is the Reaction Rate Reaction rate: the changes in concentrations of reactants or products per unit time. For the general reaction A → B, we measure the concentration of A at t 1 and at t 2: Rate = change in concentration of A change in time =- conc A 2 - conc A 1 t 2 - t 1 =- “[xxx]” = concentration in moles per liter. “-” : because the concentration of A is decreasing. This gives the rate a positive value. 16 -8 [A] t
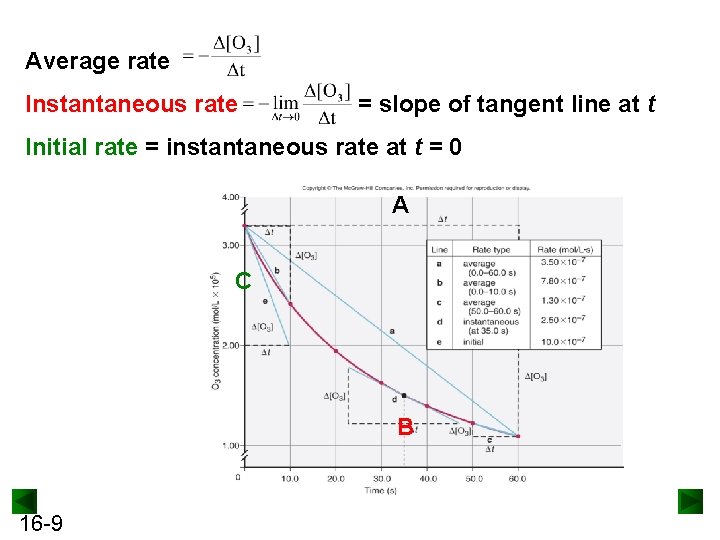
Average rate Instantaneous rate = slope of tangent line at t Initial rate = instantaneous rate at t = 0 A C B 16 -9
![Question point A or B has the higher O 3 has higher Question: point A or B? ______ has the higher [O 3] ______ has higher](https://slidetodoc.com/presentation_image_h/0160eece20d4f5f28f0b0af4406d75c5/image-10.jpg)
Question: point A or B? ______ has the higher [O 3] ______ has higher reaction rate After reaction begins, the rate (increase or decrease) A B 16 -10
![Plots of reactant and product vs time C 2 H 4 O 3 Plots of [reactant] and [product] vs. time. C 2 H 4 + O 3](https://slidetodoc.com/presentation_image_h/0160eece20d4f5f28f0b0af4406d75c5/image-11.jpg)
Plots of [reactant] and [product] vs. time. C 2 H 4 + O 3 → C 2 H 4 O + O 2 [O 2] increases just as fast as [C 2 H 4] decreases. Rate = - b = [C 2 H 4] t [C 2 H 4 O] t == [O 3] t [O 2] t Quick Question: a or b? a. A Which has higher D[O 2]? Which has higher reaction rate? Key: b; a 16 -11
![Plots of reactant and product vs time H 2 I 2 2 Plots of [reactant] and [product] vs. time. H 2 + I 2 → 2](https://slidetodoc.com/presentation_image_h/0160eece20d4f5f28f0b0af4406d75c5/image-12.jpg)
Plots of [reactant] and [product] vs. time. H 2 + I 2 → 2 HI [HI] increases twice as fast as [H 2] decreases. Rate = - Rate = [H 2] t [IH] t =- = -2 [I 2] t [H 2] t [HI] 1 = t 2 = -2 [I 2] t The expression for the rate of a reaction and its numerical value depend on which substance serves as the reference. 16 -12
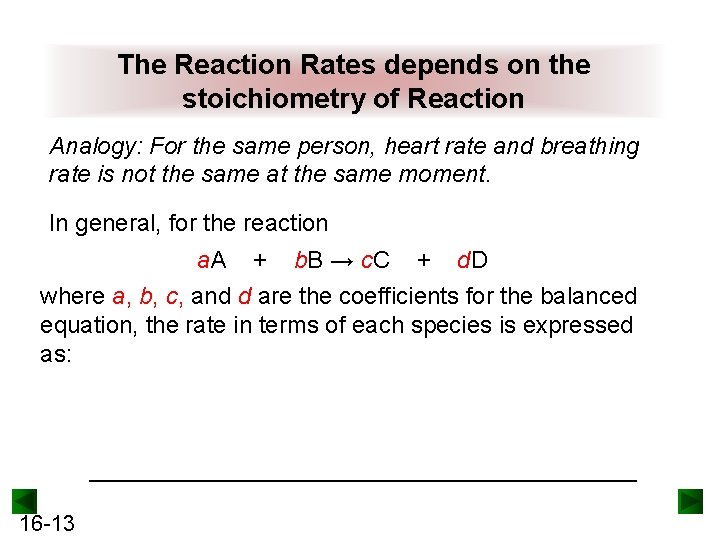
The Reaction Rates depends on the stoichiometry of Reaction Analogy: For the same person, heart rate and breathing rate is not the same at the same moment. In general, for the reaction a. A + b. B → c. C + d. D where a, b, c, and d are the coefficients for the balanced equation, the rate in terms of each species is expressed as: _____________________ 16 -13
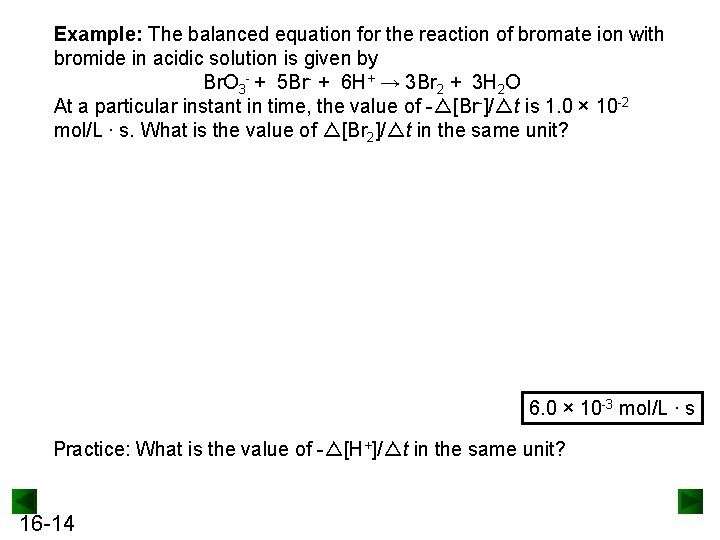
Example: The balanced equation for the reaction of bromate ion with bromide in acidic solution is given by Br. O 3 - + 5 Br- + 6 H+ → 3 Br 2 + 3 H 2 O At a particular instant in time, the value of -△[Br-]/△t is 1. 0 × 10 -2 mol/L ∙ s. What is the value of △[Br 2]/△t in the same unit? 6. 0 × 10 -3 mol/L ∙ s Practice: What is the value of -△[H+]/△t in the same unit? 16 -14
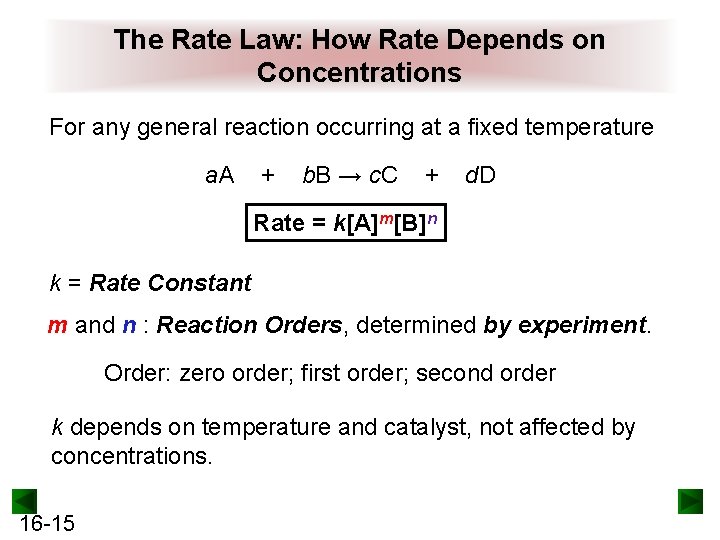
The Rate Law: How Rate Depends on Concentrations For any general reaction occurring at a fixed temperature a. A + b. B → c. C + d. D Rate = k[A]m[B]n k = Rate Constant m and n : Reaction Orders, determined by experiment. Order: zero order; first order; second order k depends on temperature and catalyst, not affected by concentrations. 16 -15
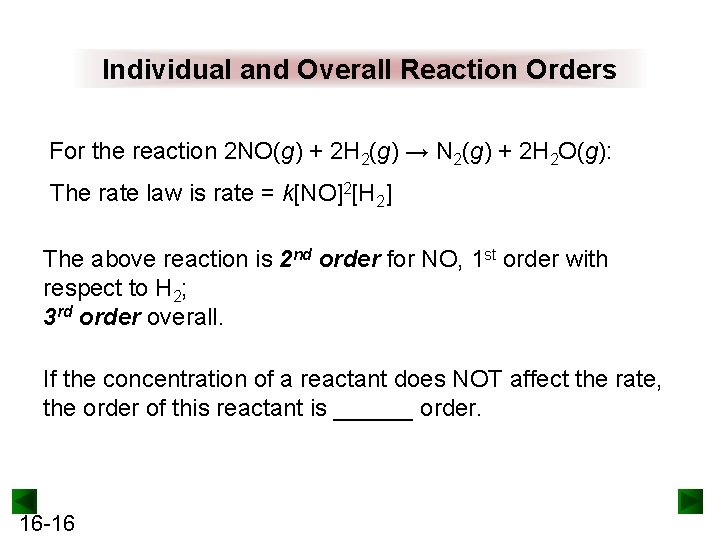
Individual and Overall Reaction Orders For the reaction 2 NO(g) + 2 H 2(g) → N 2(g) + 2 H 2 O(g): The rate law is rate = k[NO]2[H 2] The above reaction is 2 nd order for NO, 1 st order with respect to H 2; 3 rd order overall. If the concentration of a reactant does NOT affect the rate, the order of this reactant is ______ order. 16 -16
![Plots of Reactant concentration A vs Time 16 17 Plots of Reactant concentration, [A], vs. Time 16 -17](https://slidetodoc.com/presentation_image_h/0160eece20d4f5f28f0b0af4406d75c5/image-17.jpg)
Plots of Reactant concentration, [A], vs. Time 16 -17
![Plots of Rate vs Reactant Concentration A 16 18 Plots of Rate vs. Reactant Concentration [A] 16 -18](https://slidetodoc.com/presentation_image_h/0160eece20d4f5f28f0b0af4406d75c5/image-18.jpg)
Plots of Rate vs. Reactant Concentration [A] 16 -18
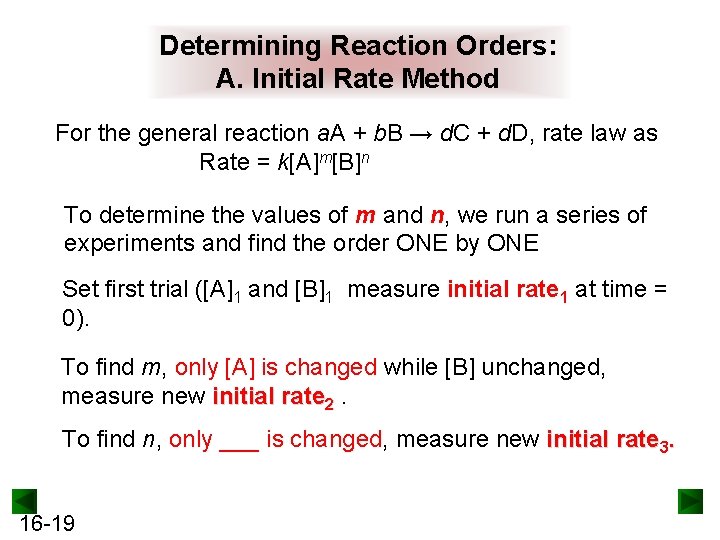
Determining Reaction Orders: A. Initial Rate Method For the general reaction a. A + b. B → d. C + d. D, rate law as Rate = k[A]m[B]n To determine the values of m and n, we run a series of experiments and find the order ONE by ONE Set first trial ([A]1 and [B]1 measure initial rate 1 at time = 0). To find m, only [A] is changed while [B] unchanged, measure new initial rate 2. To find n, only ___ is changed, measure new initial rate 3. 16 -19
![Initial Rate Method to Determine the order Experiment Initial Rate molLs Initial A molL Initial Rate Method to Determine the order Experiment Initial Rate (mol/L·s) Initial [A] (mol/L)](https://slidetodoc.com/presentation_image_h/0160eece20d4f5f28f0b0af4406d75c5/image-20.jpg)
Initial Rate Method to Determine the order Experiment Initial Rate (mol/L·s) Initial [A] (mol/L) Initial [B] (mol/L) 1 1. 75 x 10 -3 2. 50 x 10 -1 3. 00 x 10 -1 2 3. 50 x 10 -3 5. 00 x 10 -1 3 3. 95 x 10 -3 2. 50 x 10 -1 4. 50 x 10 -1 The mathematical way to find the order: order = _______ 16 -20
![Finding the order with respect to A Rate kAmBn Trial Initial Rate molLs Finding the order with respect to A Rate = k[A]m[B]n Trial Initial Rate (mol/L·s)](https://slidetodoc.com/presentation_image_h/0160eece20d4f5f28f0b0af4406d75c5/image-21.jpg)
Finding the order with respect to A Rate = k[A]m[B]n Trial Initial Rate (mol/L·s) Initial [A] (mol/L) Initial [B] (mol/L) 1 1. 75 x 10 -3 2. 50 x 10 -1 3. 00 x 10 -1 2 3. 50 x 10 -3 5. 00 x 10 -1 3 3. 95 x 10 -3 2. 50 x 10 -1 4. 50 x 10 -1 compare Trial# 1 and ___, where [B] is kept constant but [A] changes: m= 16 -21
![Practice Find the order with respect to B Rate kAmBn Trial Initial Rate Practice: Find the order with respect to B Rate = k[A]m[B]n Trial Initial Rate](https://slidetodoc.com/presentation_image_h/0160eece20d4f5f28f0b0af4406d75c5/image-22.jpg)
Practice: Find the order with respect to B Rate = k[A]m[B]n Trial Initial Rate (mol/L·s) Initial [A] (mol/L) Initial [B] (mol/L) 1 1. 75 x 10 -3 2. 50 x 10 -1 3. 00 x 10 -1 2 3. 50 x 10 -3 5. 00 x 10 -1 3 3. 95 x 10 -3 2. 50 x 10 -1 4. 50 x 10 -1 compare Trial# 1 and ___, where [A] is kept constant but [B] changes: n= 16 -22
![Practice Finding the rate constant k Initial Rate Trial molLs Initial A molL Practice: Finding the rate constant k Initial Rate = Trial (mol/L·s) Initial [A] (mol/L)](https://slidetodoc.com/presentation_image_h/0160eece20d4f5f28f0b0af4406d75c5/image-23.jpg)
Practice: Finding the rate constant k Initial Rate = Trial (mol/L·s) Initial [A] (mol/L) 1 1. 75 x 10 -3 2. 50 x 10 -1 3. 00 x 10 -1 2 3. 53 x 10 -3 5. 00 x 10 -1 3 3. 92 x 10 -3 2. 50 x 10 -1 4. 50 x 10 -1 k[A]1[B]2 Initial [B] (mol/L) Choose any trial to determine the rate constant k k= _____ Your k = _________ -2 2 -1 16 -23 Trial 1: k = 0. 0778 mol L s
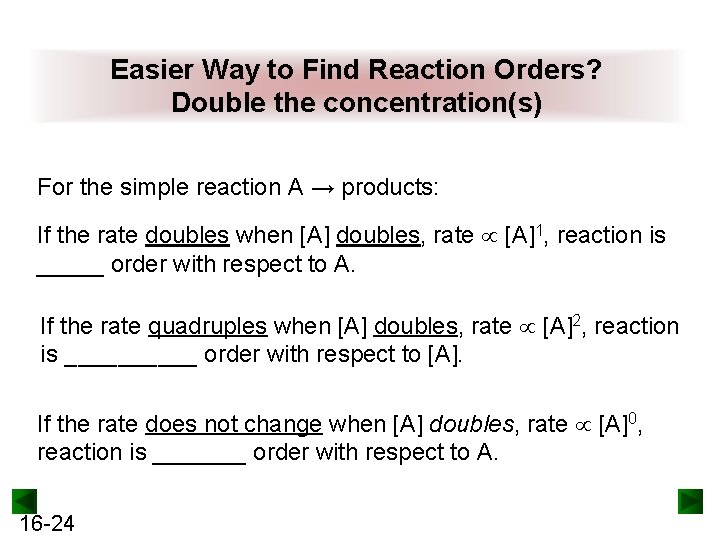
Easier Way to Find Reaction Orders? Double the concentration(s) For the simple reaction A → products: If the rate doubles when [A] doubles, rate [A]1, reaction is _____ order with respect to A. If the rate quadruples when [A] doubles, rate [A]2, reaction is _____ order with respect to [A]. If the rate does not change when [A] doubles, rate [A]0, reaction is _______ order with respect to A. 16 -24
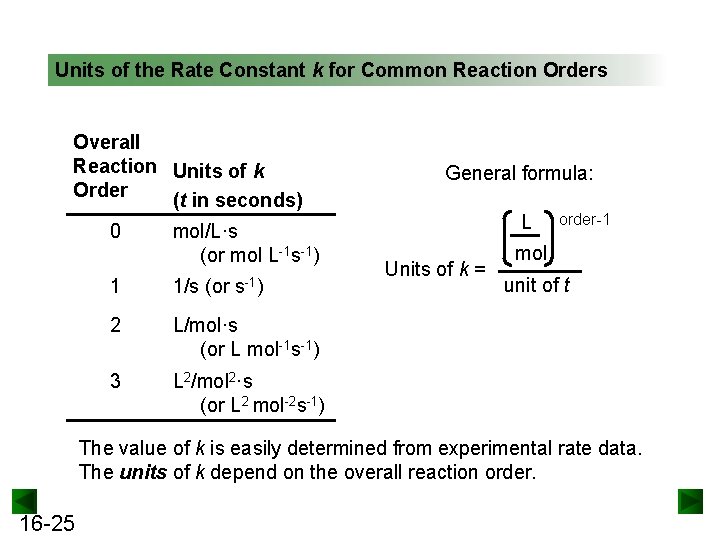
Units of the Rate Constant k for Common Reaction Orders Overall Reaction Units of k Order (t in seconds) 0 mol/L·s (or mol L-1 s-1) 1 1/s (or 2 L/mol·s (or L mol-1 s-1) 3 L 2/mol 2·s (or L 2 mol-2 s-1) General formula: L Units of k = order-1 mol unit of t The value of k is easily determined from experimental rate data. The units of k depend on the overall reaction order. 16 -25
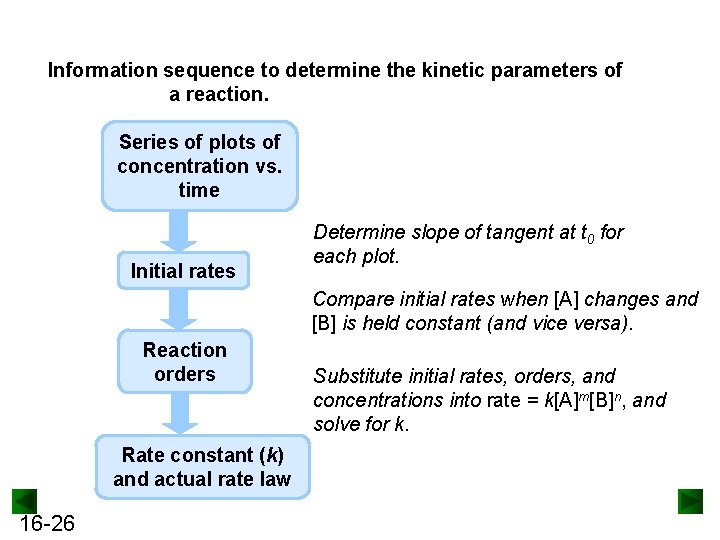
Information sequence to determine the kinetic parameters of a reaction. Series of plots of concentration vs. time Initial rates Determine slope of tangent at t 0 for each plot. Compare initial rates when [A] changes and [B] is held constant (and vice versa). Reaction orders Rate constant (k) and actual rate law 16 -26 Substitute initial rates, orders, and concentrations into rate = k[A]m[B]n, and solve for k.
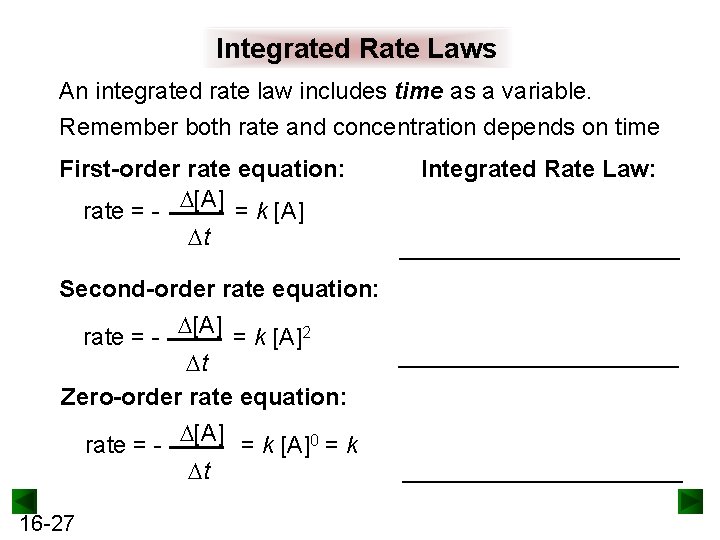
Integrated Rate Laws An integrated rate law includes time as a variable. Remember both rate and concentration depends on time First-order rate equation: rate = - [A] = k [A] t Integrated Rate Law: ___________ Second-order rate equation: rate = - [A] = k [A]2 t Zero-order rate equation: rate = - [A] = k [A]0 = k t 16 -27 _____________________
![Graphical presentation of 1 st order reaction Firstorder reaction integrated rate law A0 Graphical presentation of 1 st order reaction First-order reaction integrated rate law [A]0 =](https://slidetodoc.com/presentation_image_h/0160eece20d4f5f28f0b0af4406d75c5/image-28.jpg)
Graphical presentation of 1 st order reaction First-order reaction integrated rate law [A]0 = kt ln [A]t straight-line form ln[A]t = _______ + _____ A plot of ln[A] vs. time gives a straight line for a first-order reaction. Rate constant k = - slope 16 -28
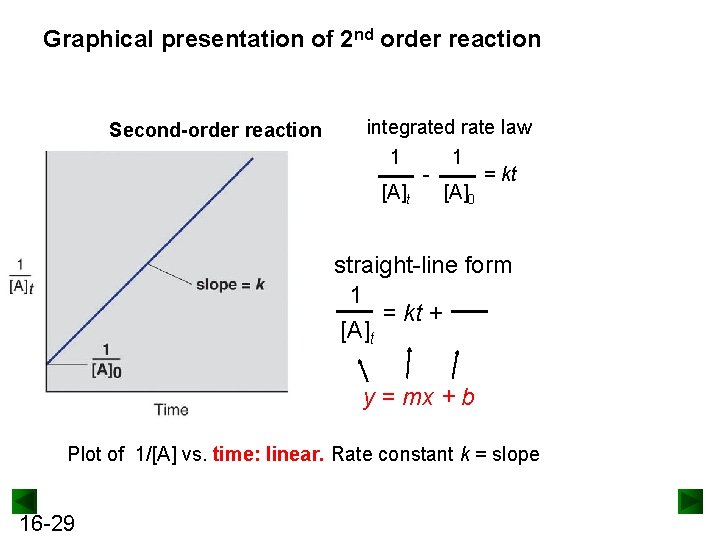
Graphical presentation of 2 nd order reaction Second-order reaction integrated rate law 1 1 = kt [A]0 straight-line form 1 = kt + [A]t y = mx + b Plot of 1/[A] vs. time: linear. Rate constant k = slope 16 -29
![Graphical presentation of zero order reaction Zeroorder reaction integrated rate law At A0 Graphical presentation of zero order reaction Zero-order reaction integrated rate law [A]t - [A]0](https://slidetodoc.com/presentation_image_h/0160eece20d4f5f28f0b0af4406d75c5/image-30.jpg)
Graphical presentation of zero order reaction Zero-order reaction integrated rate law [A]t - [A]0 = - kt straight-line form [A]t = _____ + ____ Plot of [A] vs. time gives a straight line for a first-order reaction. Rate constant k = - slope 16 -30
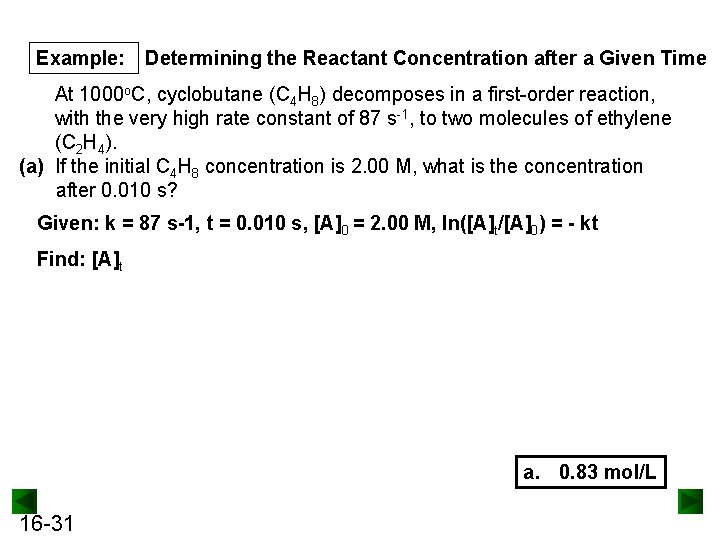
Example: Determining the Reactant Concentration after a Given Time At 1000 o. C, cyclobutane (C 4 H 8) decomposes in a first-order reaction, with the very high rate constant of 87 s-1, to two molecules of ethylene (C 2 H 4). (a) If the initial C 4 H 8 concentration is 2. 00 M, what is the concentration after 0. 010 s? Given: k = 87 s-1, t = 0. 010 s, [A]0 = 2. 00 M, ln([A]t/[A]0) = - kt Find: [A]t a. 0. 83 mol/L 16 -31
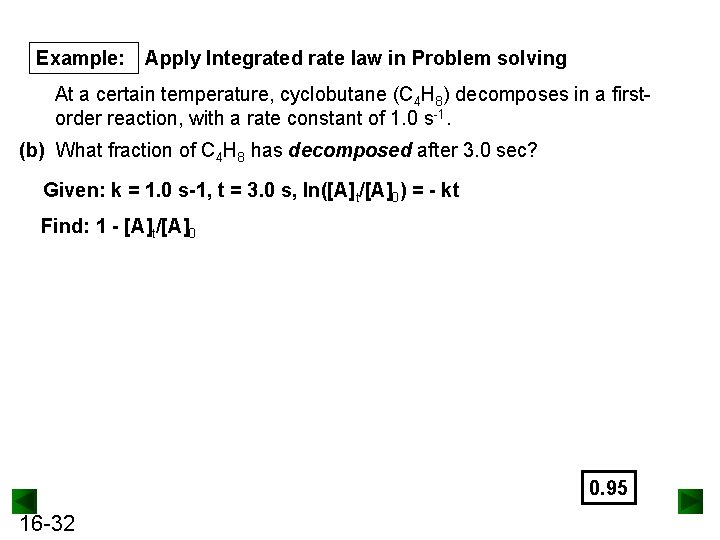
Example: Apply Integrated rate law in Problem solving At a certain temperature, cyclobutane (C 4 H 8) decomposes in a firstorder reaction, with a rate constant of 1. 0 s-1. (b) What fraction of C 4 H 8 has decomposed after 3. 0 sec? Given: k = 1. 0 s-1, t = 3. 0 s, ln([A]t/[A]0) = - kt Find: 1 - [A]t/[A]0 0. 95 16 -32
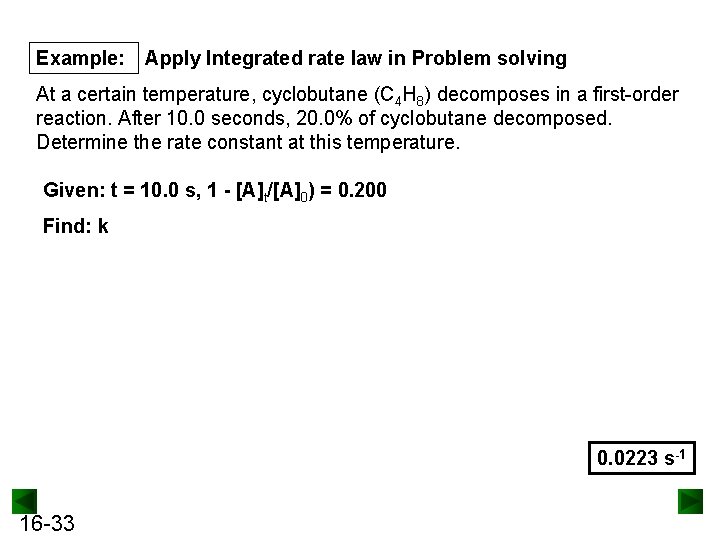
Example: Apply Integrated rate law in Problem solving At a certain temperature, cyclobutane (C 4 H 8) decomposes in a first-order reaction. After 10. 0 seconds, 20. 0% of cyclobutane decomposed. Determine the rate constant at this temperature. Given: t = 10. 0 s, 1 - [A]t/[A]0) = 0. 200 Find: k 0. 0223 s-1 16 -33
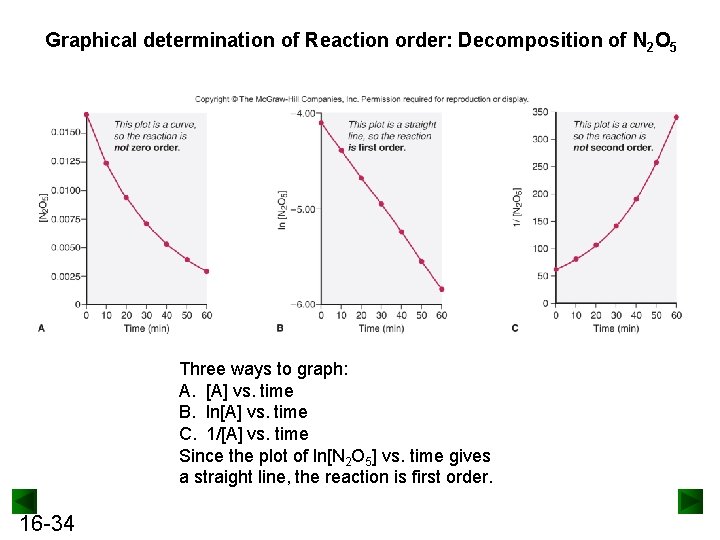
Graphical determination of Reaction order: Decomposition of N 2 O 5 Three ways to graph: A. [A] vs. time B. ln[A] vs. time C. 1/[A] vs. time Since the plot of ln[N 2 O 5] vs. time gives a straight line, the reaction is first order. 16 -34
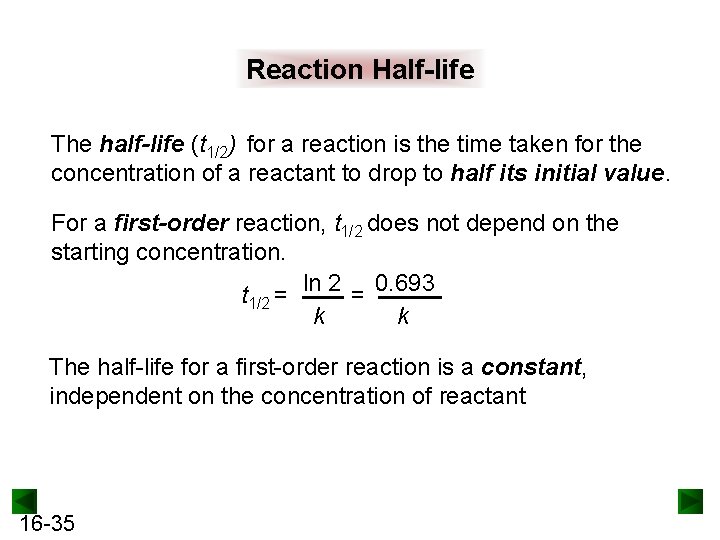
Reaction Half-life The half-life (t 1/2) for a reaction is the time taken for the concentration of a reactant to drop to half its initial value. For a first-order reaction, t 1/2 does not depend on the starting concentration. t 1/2 = ln 2 = 0. 693 k k The half-life for a first-order reaction is a constant, independent on the concentration of reactant 16 -35
![N 2 O 5 vs time for three reaction halflives for a firstorder process [N 2 O 5] vs. time for three reaction half-lives. for a first-order process](https://slidetodoc.com/presentation_image_h/0160eece20d4f5f28f0b0af4406d75c5/image-36.jpg)
[N 2 O 5] vs. time for three reaction half-lives. for a first-order process t 1/2 = 16 -36 ln 2 k = 0. 693 k
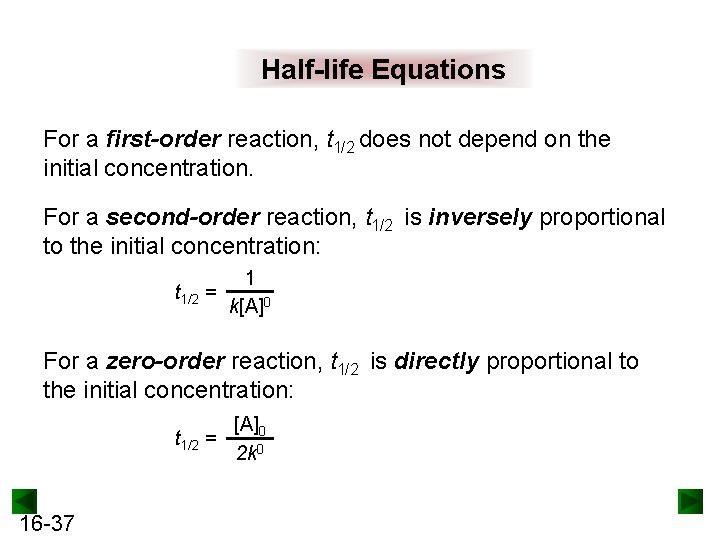
Half-life Equations For a first-order reaction, t 1/2 does not depend on the initial concentration. For a second-order reaction, t 1/2 is inversely proportional to the initial concentration: t 1/2 = 1 k[A]0 For a zero-order reaction, t 1/2 is directly proportional to the initial concentration: [A]0 t 1/2 = 2 k 0 16 -37
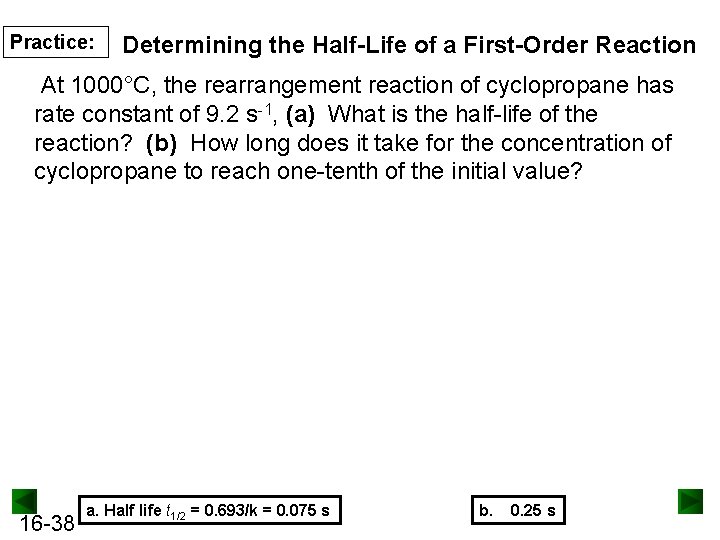
Practice: Determining the Half-Life of a First-Order Reaction At 1000°C, the rearrangement reaction of cyclopropane has rate constant of 9. 2 s-1, (a) What is the half-life of the reaction? (b) How long does it take for the concentration of cyclopropane to reach one-tenth of the initial value? 16 -38 a. Half life t 1/2 = 0. 693/k = 0. 075 s b. 0. 25 s
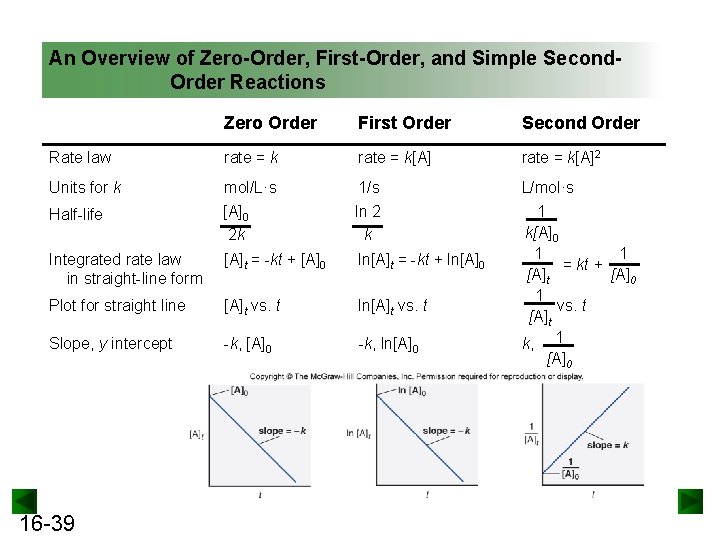
An Overview of Zero-Order, First-Order, and Simple Second. Order Reactions Zero Order First Order Second Order Rate law rate = k[A]2 Units for k mol/L·s 1/s Half-life [A]0 2 k ln 2 k Integrated rate law in straight-line form [A]t = -kt + [A]0 ln[A]t = -kt + ln[A]0 Plot for straight line [A]t vs. t ln[A]t vs. t Slope, y intercept -k, [A]0 -k, ln[A]0 L/mol·s 1 k[A]0 1 1 = kt + [A]t [A]0 1 vs. t [A]t k, 1 [A]0 16 -39
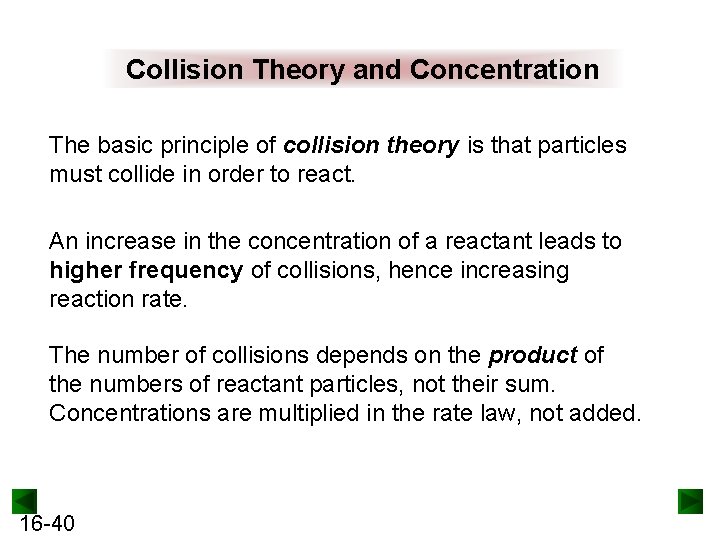
Collision Theory and Concentration The basic principle of collision theory is that particles must collide in order to react. An increase in the concentration of a reactant leads to higher frequency of collisions, hence increasing reaction rate. The number of collisions depends on the product of the numbers of reactant particles, not their sum. Concentrations are multiplied in the rate law, not added. 16 -40
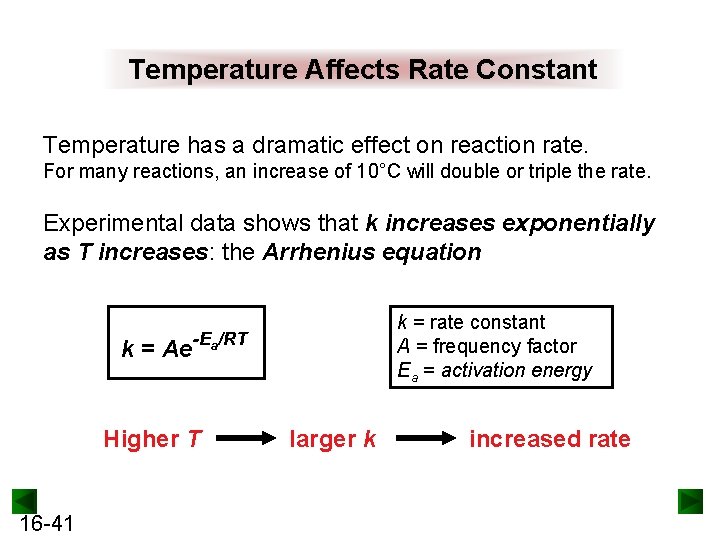
Temperature Affects Rate Constant Temperature has a dramatic effect on reaction rate. For many reactions, an increase of 10°C will double or triple the rate. Experimental data shows that k increases exponentially as T increases: the Arrhenius equation k = rate constant A = frequency factor Ea = activation energy k = Ae-Ea/RT Higher T 16 -41 larger k increased rate
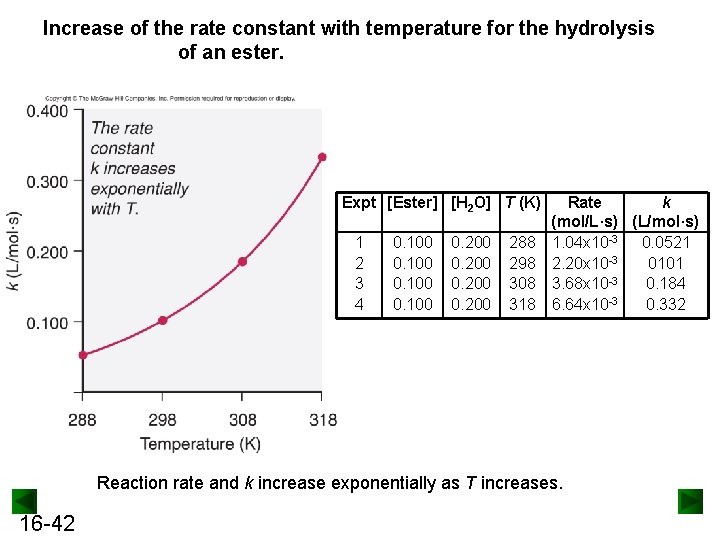
Increase of the rate constant with temperature for the hydrolysis of an ester. Expt [Ester] [H 2 O] T (K) 1 2 3 4 0. 100 0. 200 288 298 308 318 Rate k (mol/L·s) (L/mol·s) 1. 04 x 10 -3 0. 0521 2. 20 x 10 -3 0101 3. 68 x 10 -3 0. 184 6. 64 x 10 -3 0. 332 Reaction rate and k increase exponentially as T increases. 16 -42
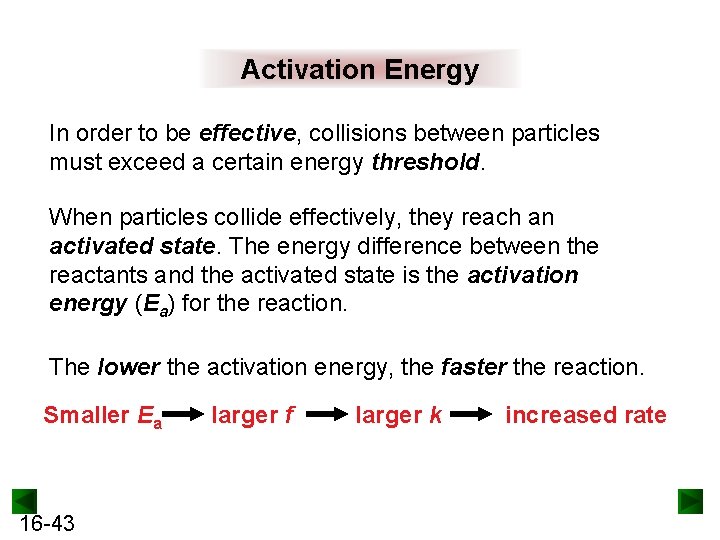
Activation Energy In order to be effective, collisions between particles must exceed a certain energy threshold. When particles collide effectively, they reach an activated state. The energy difference between the reactants and the activated state is the activation energy (Ea) for the reaction. The lower the activation energy, the faster the reaction. Smaller Ea 16 -43 larger f larger k increased rate
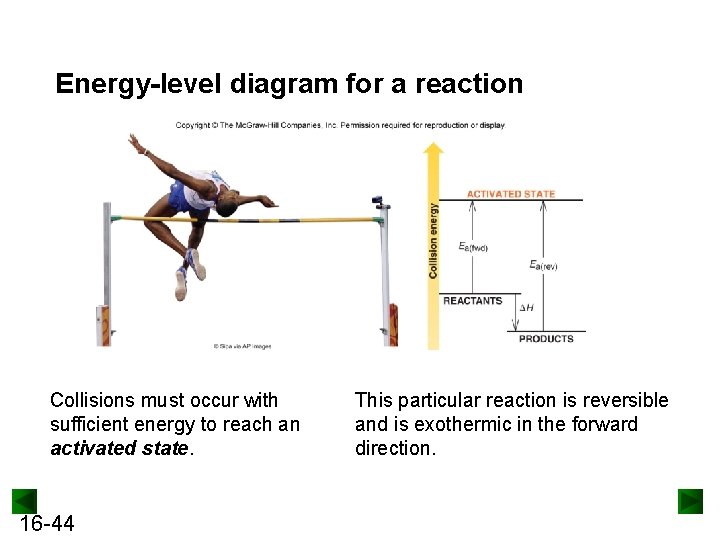
Energy-level diagram for a reaction Collisions must occur with sufficient energy to reach an activated state. 16 -44 This particular reaction is reversible and is exothermic in the forward direction.
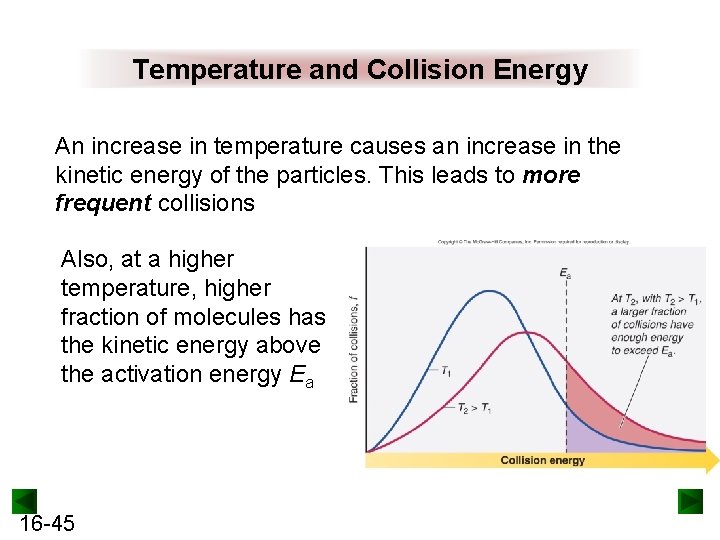
Temperature and Collision Energy An increase in temperature causes an increase in the kinetic energy of the particles. This leads to more frequent collisions Also, at a higher temperature, higher fraction of molecules has the kinetic energy above the activation energy Ea 16 -45
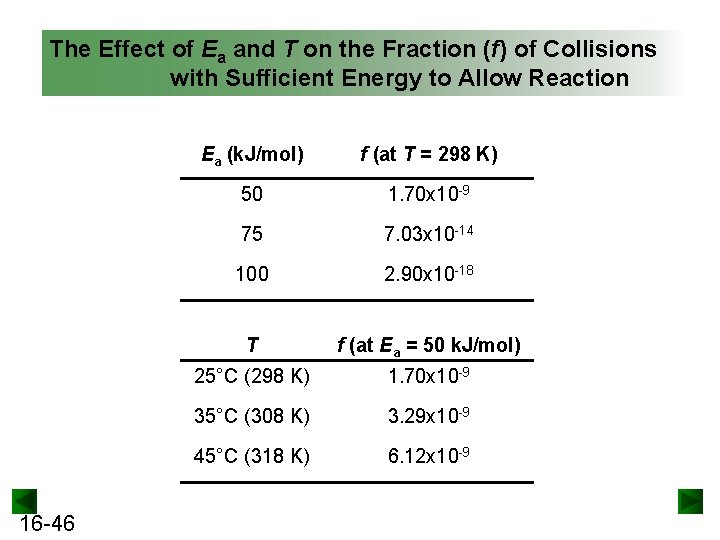
The Effect of Ea and T on the Fraction (f) of Collisions with Sufficient Energy to Allow Reaction 16 -46 Ea (k. J/mol) f (at T = 298 K) 50 1. 70 x 10 -9 75 7. 03 x 10 -14 100 2. 90 x 10 -18 T f (at Ea = 50 k. J/mol) 25°C (298 K) 1. 70 x 10 -9 35°C (308 K) 3. 29 x 10 -9 45°C (318 K) 6. 12 x 10 -9
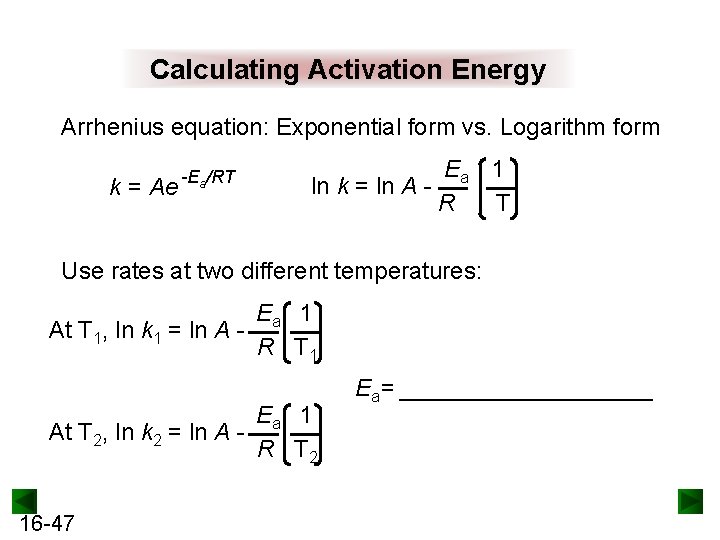
Calculating Activation Energy Arrhenius equation: Exponential form vs. Logarithm form k = Ae -Ea/RT Ea 1 ln k = ln A R T Use rates at two different temperatures: Ea 1 At T 1, ln k 1 = ln A R T 1 Ea 1 At T 2, ln k 2 = ln A R T 2 16 -47 Ea= __________
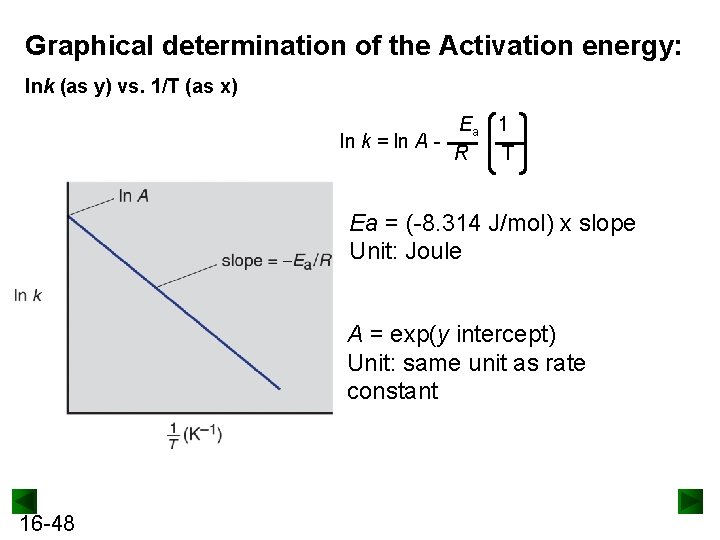
Graphical determination of the Activation energy: lnk (as y) vs. 1/T (as x) Ea 1 ln k = ln A R T Ea = (-8. 314 J/mol) x slope Unit: Joule A = exp(y intercept) Unit: same unit as rate constant 16 -48
![Find Activation Energy and A factor from graph Expt Ester H 2 O T Find Activation Energy and A factor from graph Expt [Ester] [H 2 O] T](https://slidetodoc.com/presentation_image_h/0160eece20d4f5f28f0b0af4406d75c5/image-49.jpg)
Find Activation Energy and A factor from graph Expt [Ester] [H 2 O] T (K) 1 2 3 4 0. 100 0. 200 288 298 308 318 Rate k (mol/L·s) (L/mol·s) 1. 04 x 10 -3 0. 0521 2. 20 x 10 -3 0101 3. 68 x 10 -3 0. 184 6. 64 x 10 -3 0. 332 1/T (K-1) 0 0. 00309 -0. 5 lnk -1 -1. 5 0. 00319 0. 00329 0. 00339 0. 00349 R 2 = 0. 9999 0. 00359 Ea = (-8. 314 J/mol) x slope A = exp(y intercept) -2 -2. 5 -3 -3. 5 16 -49 46. 9 k. J/mol 1. 6 x 107 L/mol s
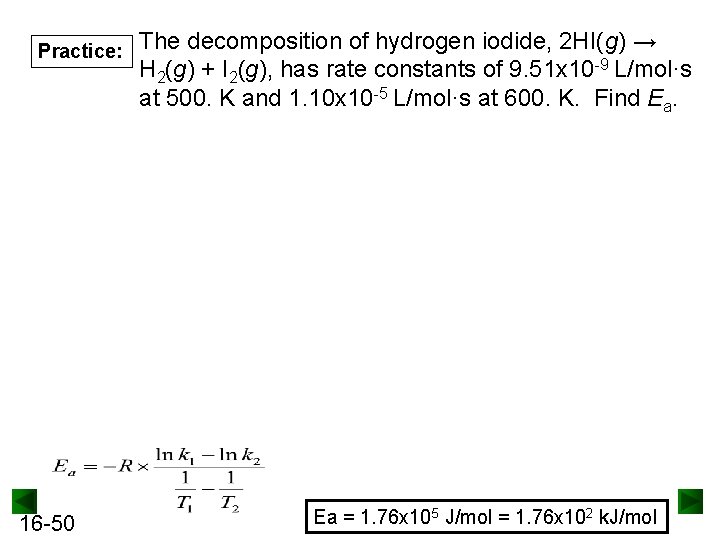
Practice: 16 -50 The decomposition of hydrogen iodide, 2 HI(g) → H 2(g) + I 2(g), has rate constants of 9. 51 x 10 -9 L/mol·s at 500. K and 1. 10 x 10 -5 L/mol·s at 600. K. Find Ea. Ea = 1. 76 x 105 J/mol = 1. 76 x 102 k. J/mol
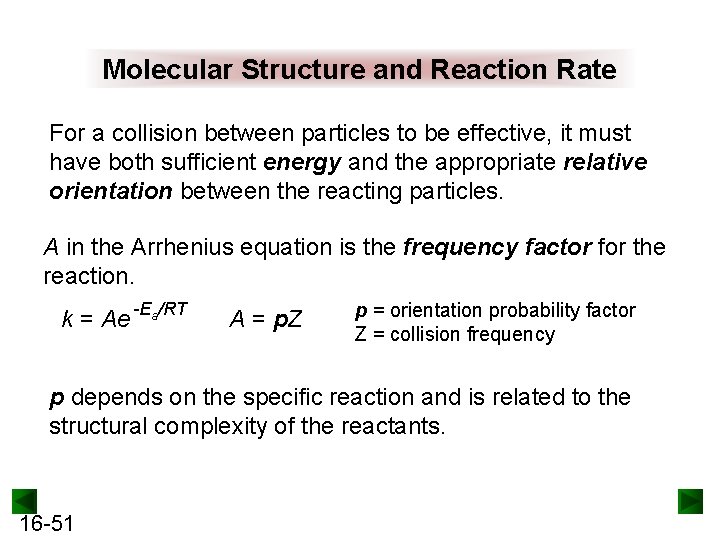
Molecular Structure and Reaction Rate For a collision between particles to be effective, it must have both sufficient energy and the appropriate relative orientation between the reacting particles. A in the Arrhenius equation is the frequency factor for the reaction. k = Ae -Ea/RT A = p. Z p = orientation probability factor Z = collision frequency p depends on the specific reaction and is related to the structural complexity of the reactants. 16 -51
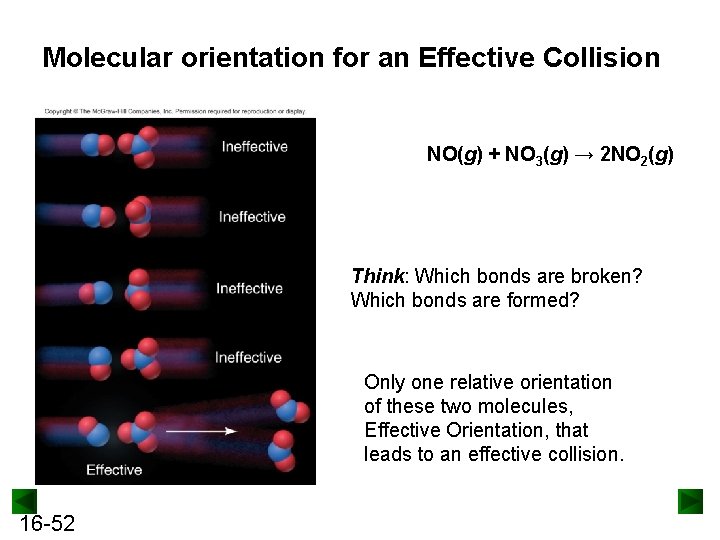
Molecular orientation for an Effective Collision NO(g) + NO 3(g) → 2 NO 2(g) Think: Which bonds are broken? Which bonds are formed? Only one relative orientation of these two molecules, Effective Orientation, that leads to an effective collision. 16 -52
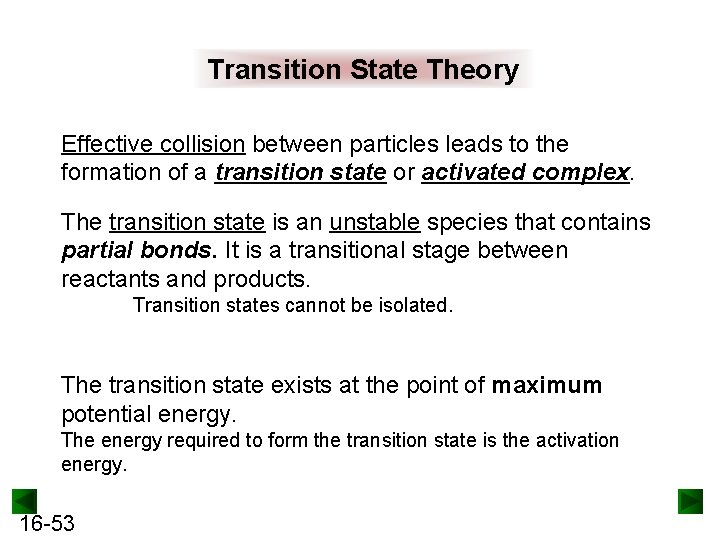
Transition State Theory Effective collision between particles leads to the formation of a transition state or activated complex. The transition state is an unstable species that contains partial bonds. It is a transitional stage between reactants and products. Transition states cannot be isolated. The transition state exists at the point of maximum potential energy. The energy required to form the transition state is the activation energy. 16 -53
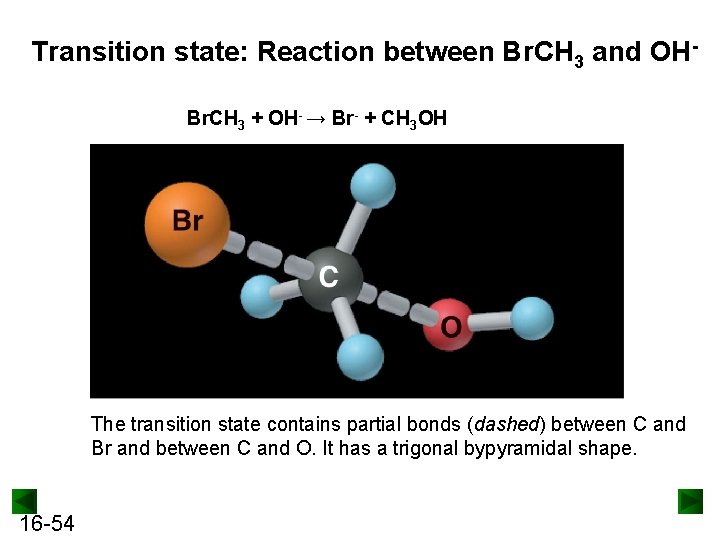
Transition state: Reaction between Br. CH 3 and OHBr. CH 3 + OH- → Br- + CH 3 OH The transition state contains partial bonds (dashed) between C and Br and between C and O. It has a trigonal bypyramidal shape. 16 -54
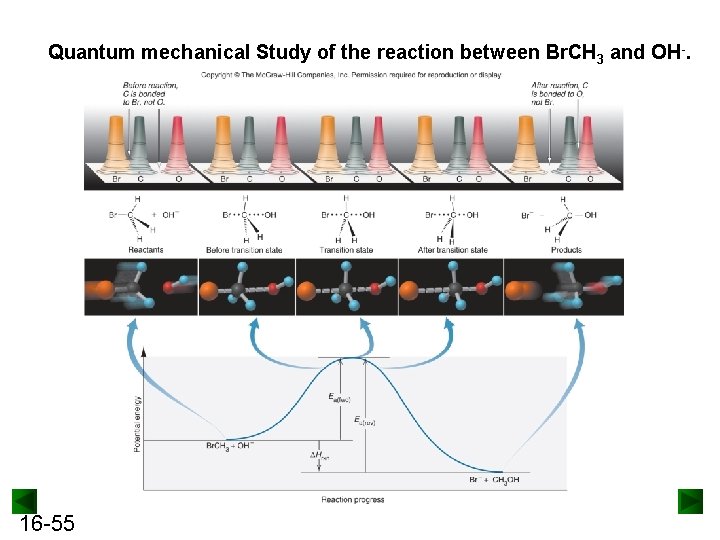
Quantum mechanical Study of the reaction between Br. CH 3 and OH-. 16 -55
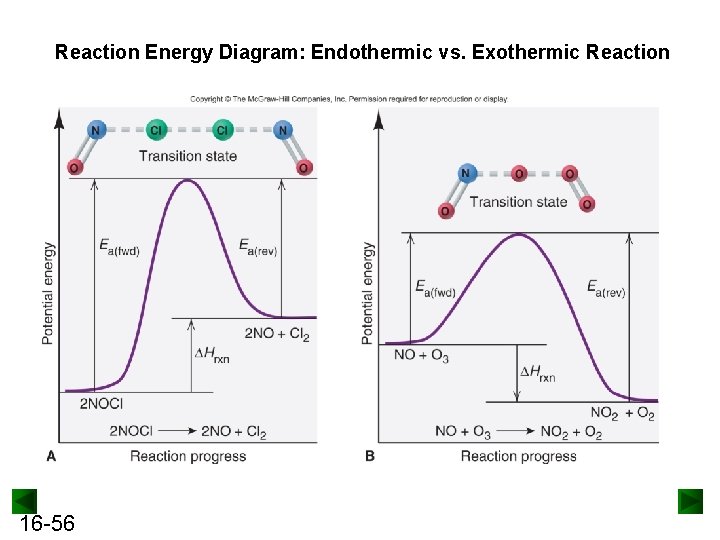
Reaction Energy Diagram: Endothermic vs. Exothermic Reaction 16 -56
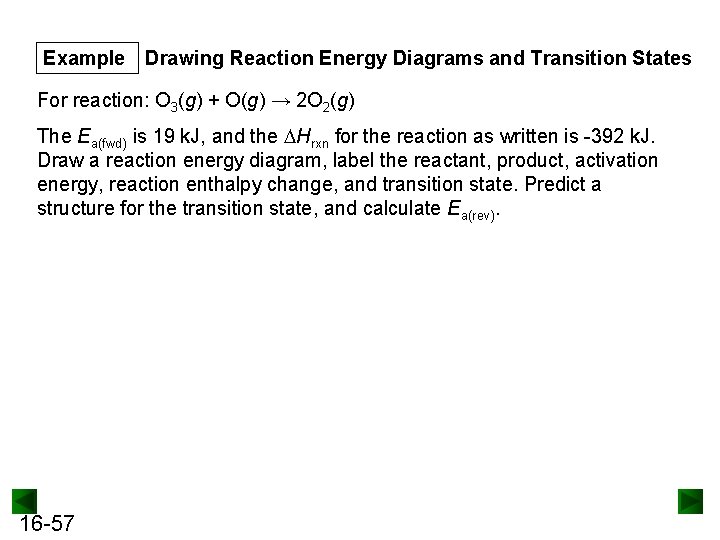
Example Drawing Reaction Energy Diagrams and Transition States For reaction: O 3(g) + O(g) → 2 O 2(g) The Ea(fwd) is 19 k. J, and the Hrxn for the reaction as written is -392 k. J. Draw a reaction energy diagram, label the reactant, product, activation energy, reaction enthalpy change, and transition state. Predict a structure for the transition state, and calculate Ea(rev). 16 -57
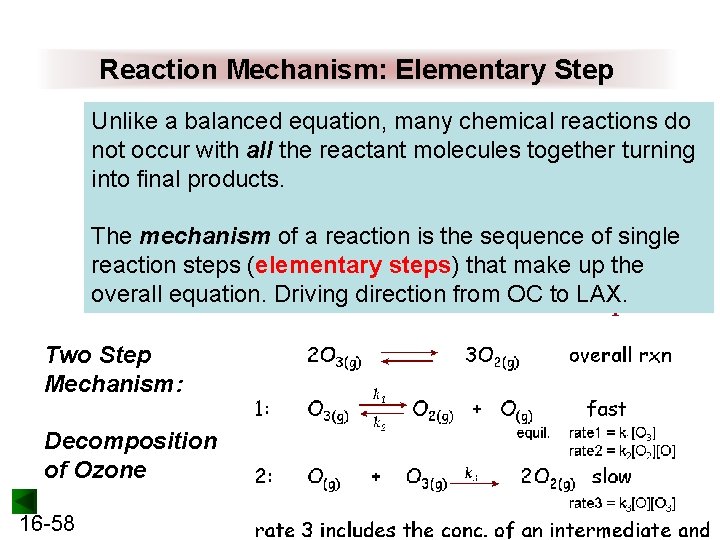
Reaction Mechanism: Elementary Step Unlike a balanced equation, many chemical reactions do not occur with all the reactant molecules together turning into final products. The mechanism of a reaction is the sequence of single reaction steps (elementary steps) that make up the overall equation. Driving direction from OC to LAX. Two Step Mechanism: Decomposition of Ozone 16 -58
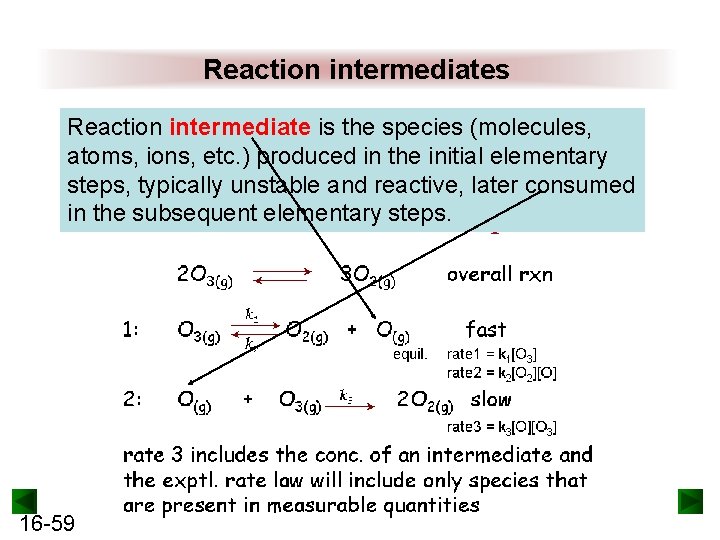
Reaction intermediates Reaction intermediate is the species (molecules, atoms, ions, etc. ) produced in the initial elementary steps, typically unstable and reactive, later consumed in the subsequent elementary steps. 16 -59
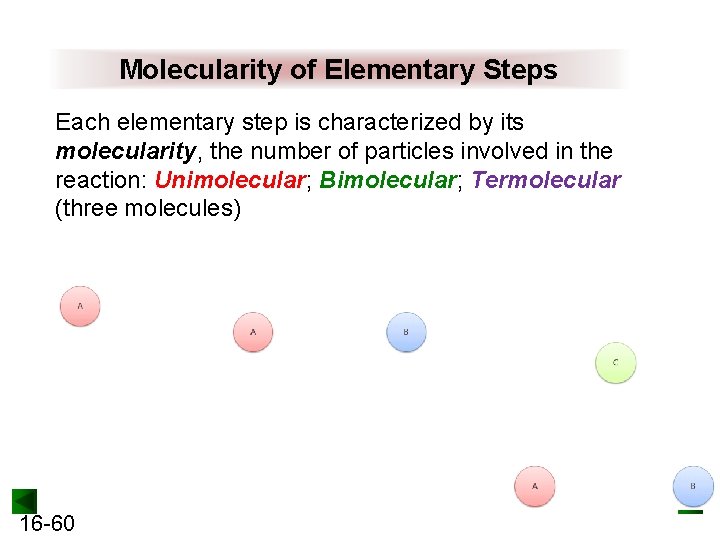
Molecularity of Elementary Steps Each elementary step is characterized by its molecularity, the number of particles involved in the reaction: Unimolecular; Bimolecular; Termolecular (three molecules) 16 -60
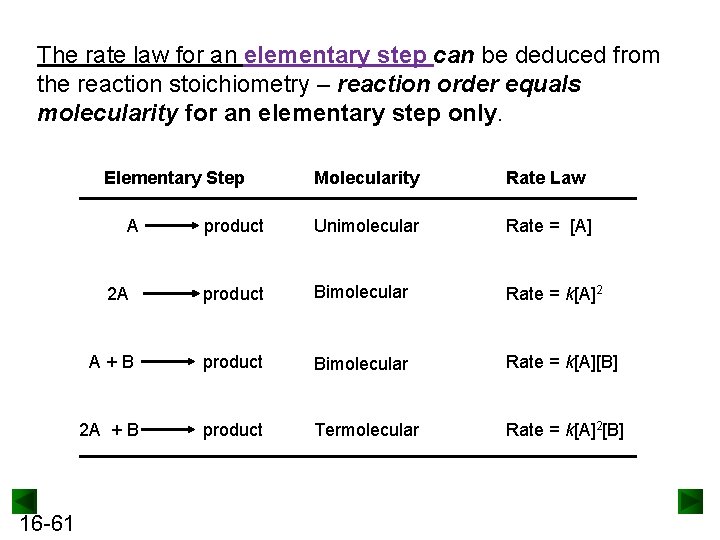
The rate law for an elementary step can be deduced from the reaction stoichiometry – reaction order equals molecularity for an elementary step only. Elementary Step Molecularity Rate Law product Unimolecular Rate = [A] 2 A product Bimolecular Rate = k[A]2 A+B product Bimolecular Rate = k[A][B] 2 A + B product Termolecular Rate = k[A]2[B] A 16 -61
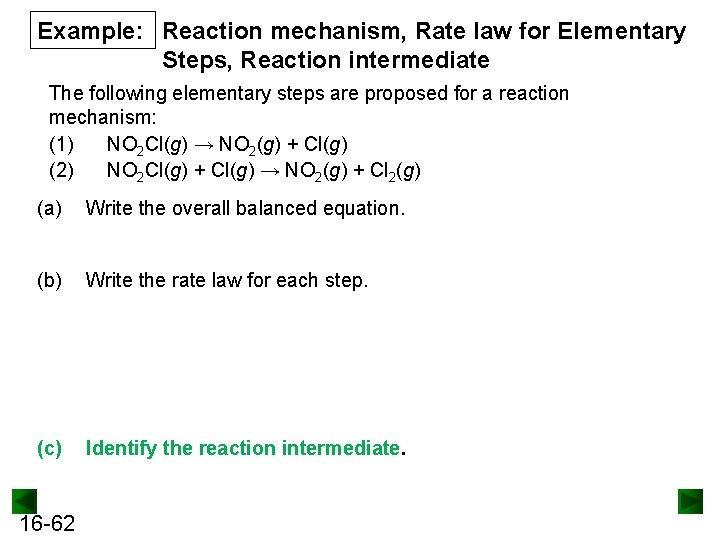
Example: Reaction mechanism, Rate law for Elementary Steps, Reaction intermediate The following elementary steps are proposed for a reaction mechanism: (1) NO 2 Cl(g) → NO 2(g) + Cl(g) (2) NO 2 Cl(g) + Cl(g) → NO 2(g) + Cl 2(g) (a) Write the overall balanced equation. (b) Write the rate law for each step. (c) Identify the reaction intermediate. 16 -62
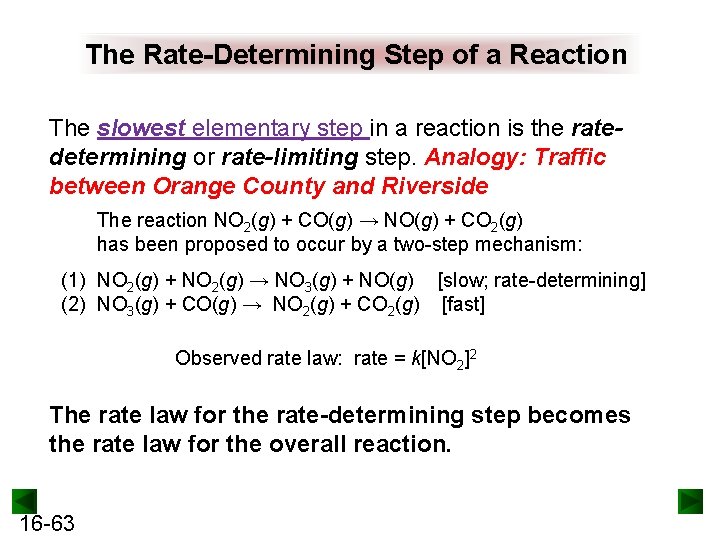
The Rate-Determining Step of a Reaction The slowest elementary step in a reaction is the ratedetermining or rate-limiting step. Analogy: Traffic between Orange County and Riverside The reaction NO 2(g) + CO(g) → NO(g) + CO 2(g) has been proposed to occur by a two-step mechanism: (1) NO 2(g) + NO 2(g) → NO 3(g) + NO(g) [slow; rate-determining] (2) NO 3(g) + CO(g) → NO 2(g) + CO 2(g) [fast] Observed rate law: rate = k[NO 2]2 The rate law for the rate-determining step becomes the rate law for the overall reaction. 16 -63
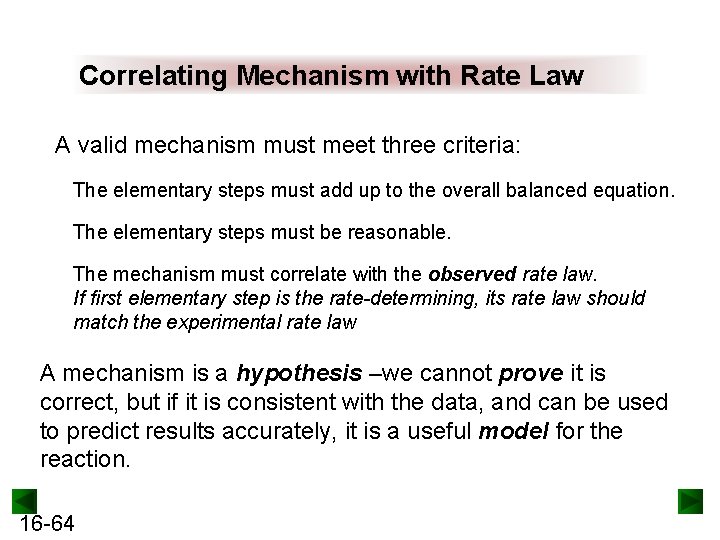
Correlating Mechanism with Rate Law A valid mechanism must meet three criteria: The elementary steps must add up to the overall balanced equation. The elementary steps must be reasonable. The mechanism must correlate with the observed rate law. If first elementary step is the rate-determining, its rate law should match the experimental rate law A mechanism is a hypothesis –we cannot prove it is correct, but if it is consistent with the data, and can be used to predict results accurately, it is a useful model for the reaction. 16 -64
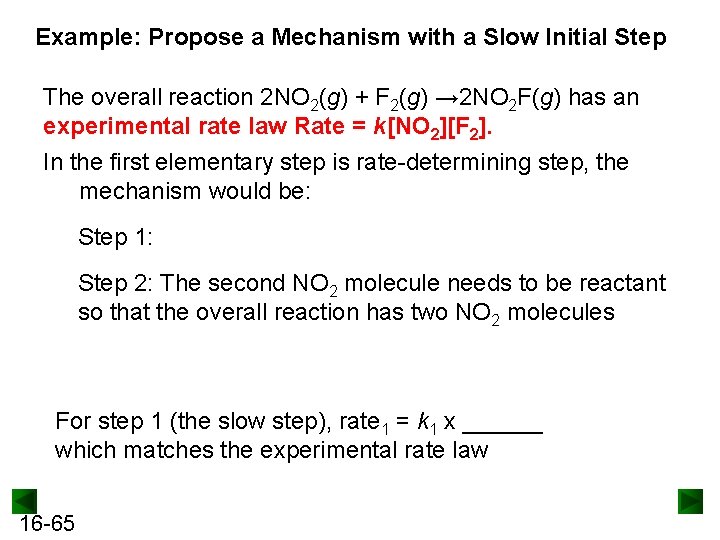
Example: Propose a Mechanism with a Slow Initial Step The overall reaction 2 NO 2(g) + F 2(g) → 2 NO 2 F(g) has an experimental rate law Rate = k[NO 2][F 2]. In the first elementary step is rate-determining step, the mechanism would be: Step 1: Step 2: The second NO 2 molecule needs to be reactant so that the overall reaction has two NO 2 molecules For step 1 (the slow step), rate 1 = k 1 x ______ which matches the experimental rate law 16 -65
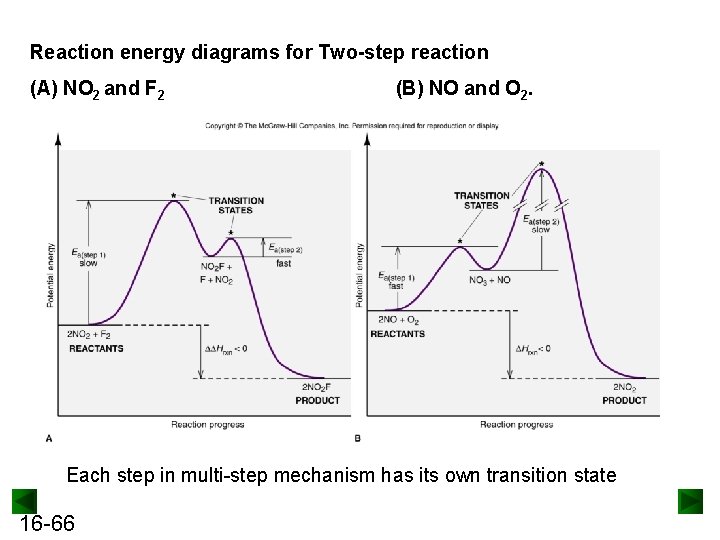
Reaction energy diagrams for Two-step reaction (A) NO 2 and F 2 (B) NO and O 2. Each step in multi-step mechanism has its own transition state 16 -66
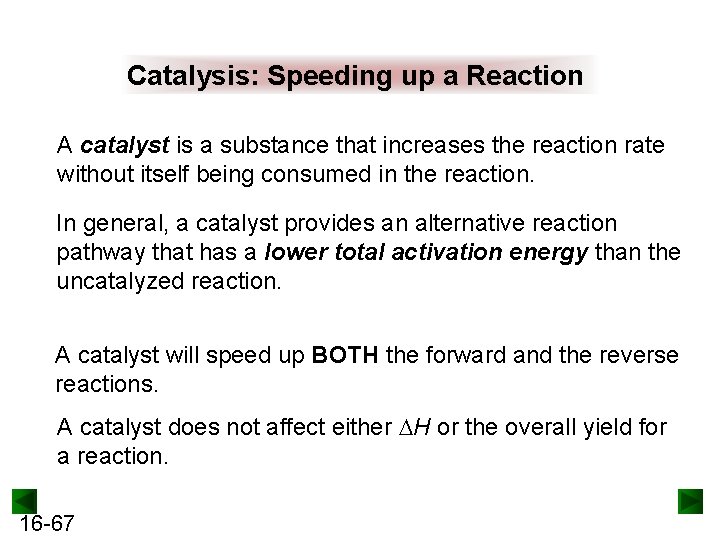
Catalysis: Speeding up a Reaction A catalyst is a substance that increases the reaction rate without itself being consumed in the reaction. In general, a catalyst provides an alternative reaction pathway that has a lower total activation energy than the uncatalyzed reaction. A catalyst will speed up BOTH the forward and the reverse reactions. A catalyst does not affect either H or the overall yield for a reaction. 16 -67
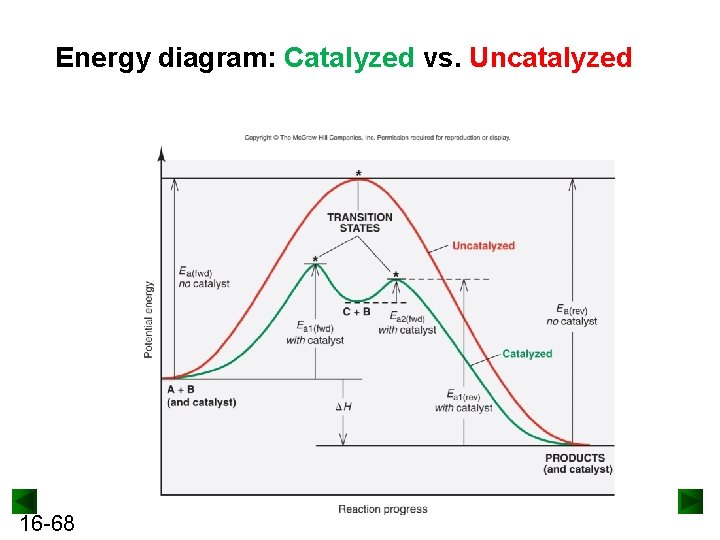
Energy diagram: Catalyzed vs. Uncatalyzed 16 -68
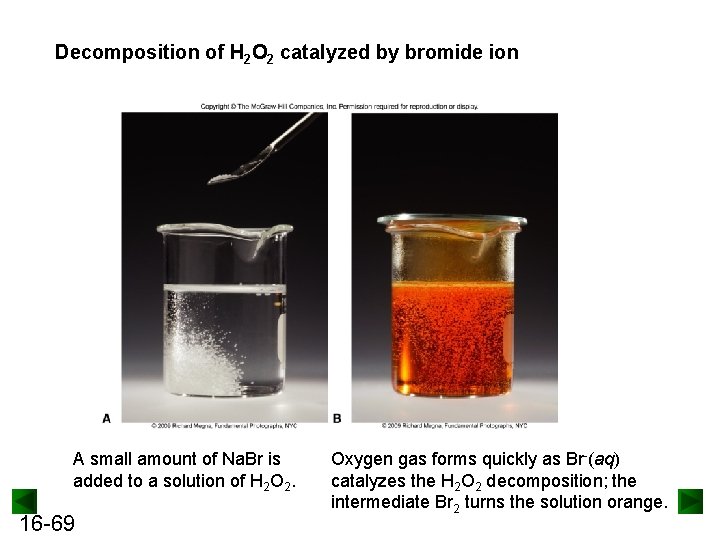
Decomposition of H 2 O 2 catalyzed by bromide ion A small amount of Na. Br is added to a solution of H 2 O 2. 16 -69 Oxygen gas forms quickly as Br-(aq) catalyzes the H 2 O 2 decomposition; the intermediate Br 2 turns the solution orange.
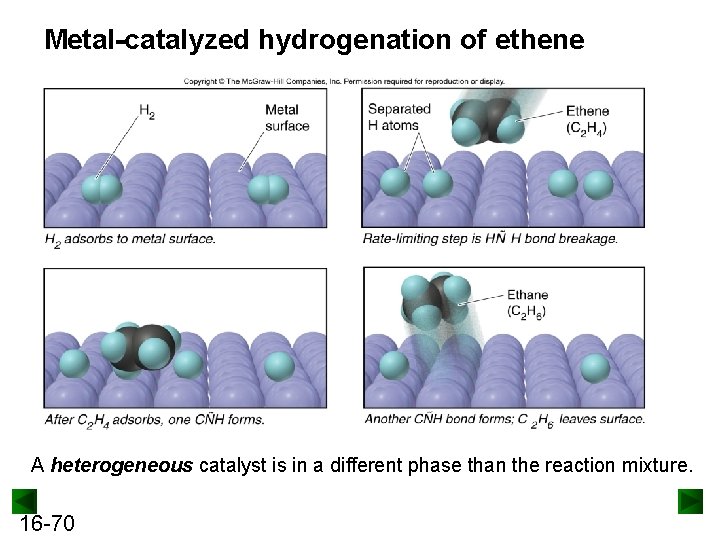
Metal-catalyzed hydrogenation of ethene A heterogeneous catalyst is in a different phase than the reaction mixture. 16 -70
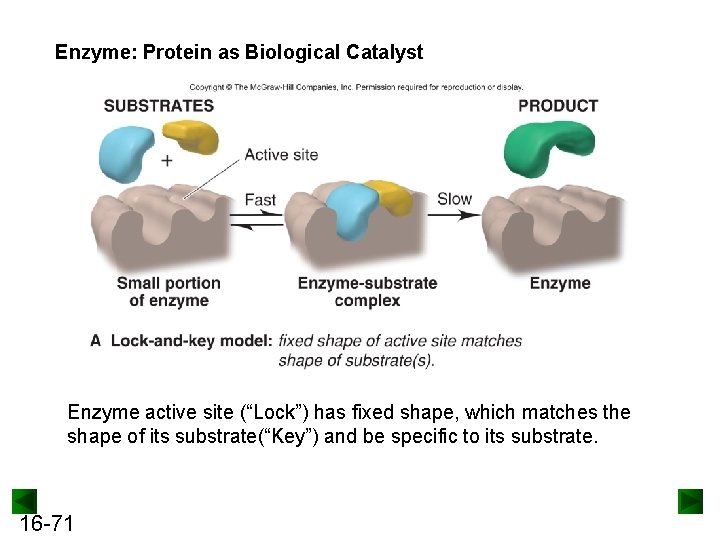
Enzyme: Protein as Biological Catalyst Enzyme active site (“Lock”) has fixed shape, which matches the shape of its substrate(“Key”) and be specific to its substrate. 16 -71
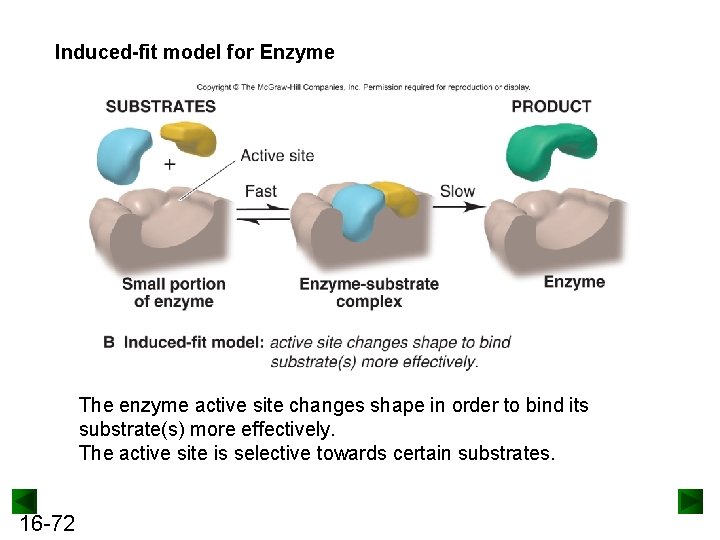
Induced-fit model for Enzyme The enzyme active site changes shape in order to bind its substrate(s) more effectively. The active site is selective towards certain substrates. 16 -72
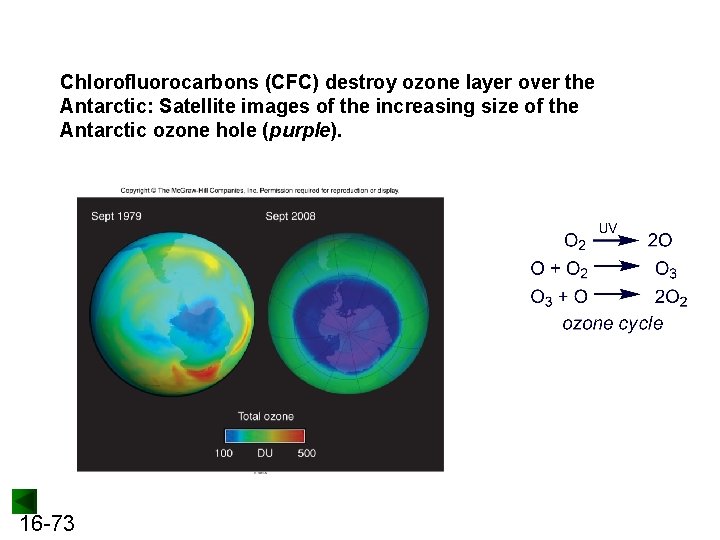
Chlorofluorocarbons (CFC) destroy ozone layer over the Antarctic: Satellite images of the increasing size of the Antarctic ozone hole (purple). 16 -73
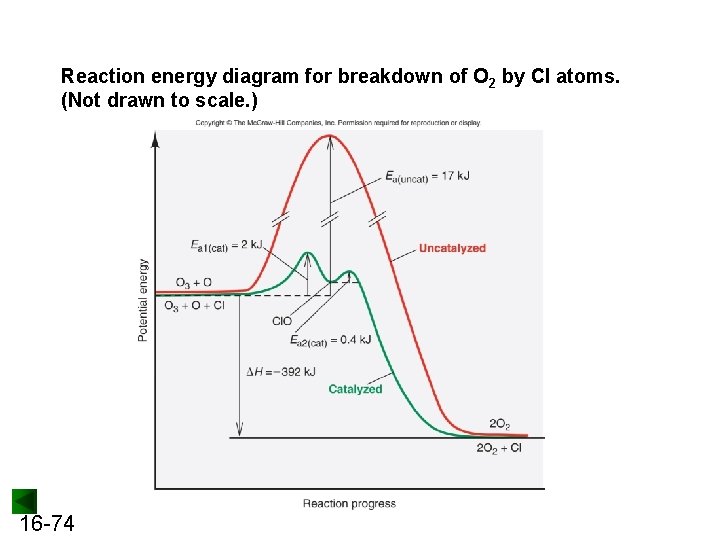
Reaction energy diagram for breakdown of O 2 by Cl atoms. (Not drawn to scale. ) 16 -74
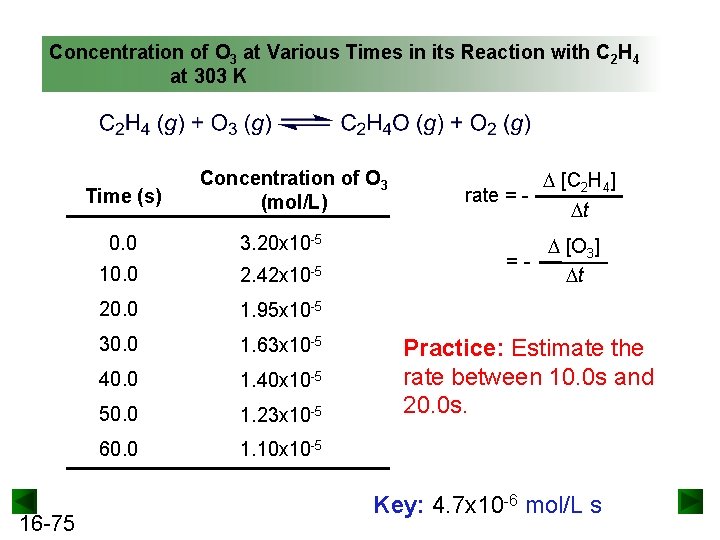
Concentration of O 3 at Various Times in its Reaction with C 2 H 4 at 303 K Time (s) 16 -75 Concentration of O 3 (mol/L) 0. 0 3. 20 x 10 -5 10. 0 2. 42 x 10 -5 20. 0 1. 95 x 10 -5 30. 0 1. 63 x 10 -5 40. 0 1. 40 x 10 -5 50. 0 1. 23 x 10 -5 60. 0 1. 10 x 10 -5 [C 2 H 4] rate = t =- [O 3] t Practice: Estimate the rate between 10. 0 s and 20. 0 s. Key: 4. 7 x 10 -6 mol/L s
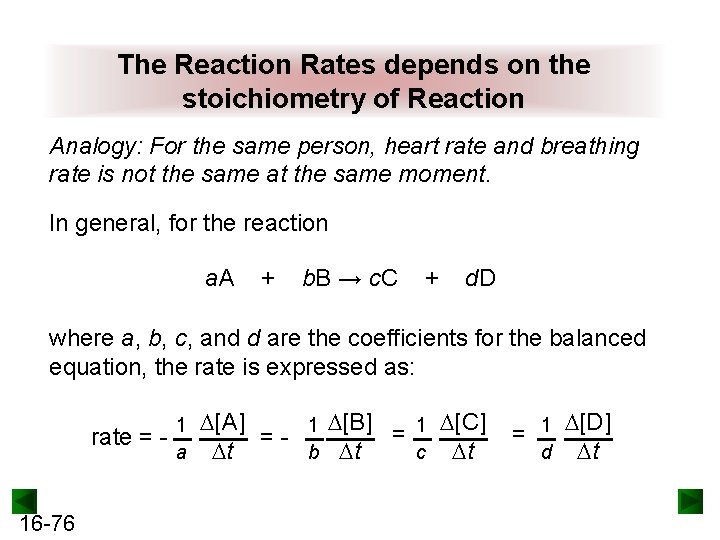
The Reaction Rates depends on the stoichiometry of Reaction Analogy: For the same person, heart rate and breathing rate is not the same at the same moment. In general, for the reaction a. A + b. B → c. C + d. D where a, b, c, and d are the coefficients for the balanced equation, the rate is expressed as: rate = - 16 -76 1 a [A] 1 [B] = 1 [C] =b t c t t = 1 [D] d t
![Rate kAmBn Finding m the order with respect to A We compare experiments Rate = k[A]m[B]n Finding m, the order with respect to A: We compare experiments](https://slidetodoc.com/presentation_image_h/0160eece20d4f5f28f0b0af4406d75c5/image-77.jpg)
Rate = k[A]m[B]n Finding m, the order with respect to A: We compare experiments 1 and 2, where [B] is kept constant but [A] doubles: Rate 2 Rate 1 = k[A] m [B] n 2 k[A] m 1 2 [B] n 1 m = [A] 2 m [A]1 = [A]2 m [A]1 m 3. 50 x 10 -3 mol/L·s 1. 75 x 10 -3 mol/L·s = 5. 00 x 10 -2 mol/L 2. 50 x 10 -2 mol/L Dividing, we get 2. 00 = (2. 00)m so m = 1 16 -77
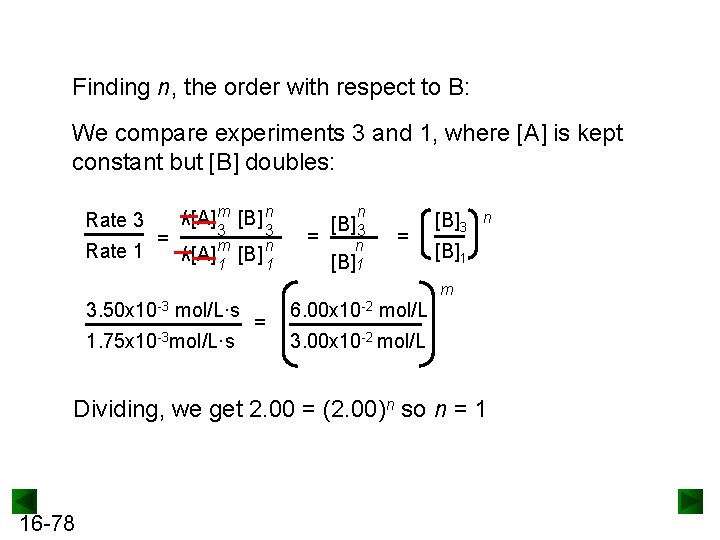
Finding n, the order with respect to B: We compare experiments 3 and 1, where [A] is kept constant but [B] doubles: Rate 3 Rate 1 = k[A] m [B] n 3 k[A] m 1 3 [B] n 1 n = [B] 3 n [B]1 = [B]3 n [B]1 m 3. 50 x 10 -3 mol/L·s 1. 75 x 10 -3 mol/L·s = 6. 00 x 10 -2 mol/L 3. 00 x 10 -2 mol/L Dividing, we get 2. 00 = (2. 00)n so n = 1 16 -78
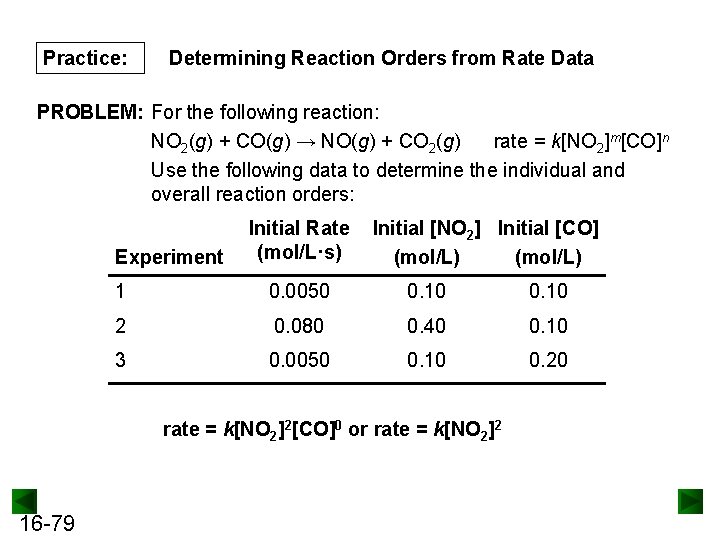
Practice: Determining Reaction Orders from Rate Data PROBLEM: For the following reaction: NO 2(g) + CO(g) → NO(g) + CO 2(g) rate = k[NO 2]m[CO]n Use the following data to determine the individual and overall reaction orders: Experiment Initial Rate (mol/L·s) Initial [NO 2] Initial [CO] (mol/L) 1 0. 0050 0. 10 2 0. 080 0. 40 0. 10 3 0. 0050 0. 10 0. 20 rate = k[NO 2]2[CO]0 or rate = k[NO 2]2 16 -79
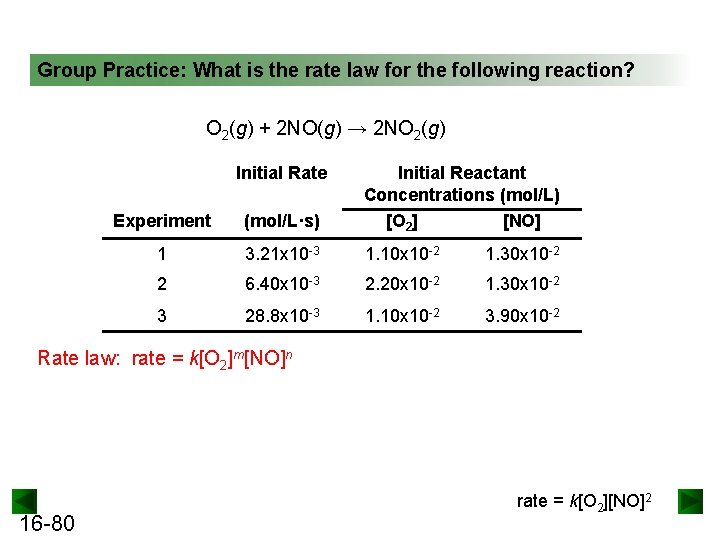
Group Practice: What is the rate law for the following reaction? O 2(g) + 2 NO(g) → 2 NO 2(g) Initial Rate Experiment (mol/L·s) Initial Reactant Concentrations (mol/L) [O 2] [NO] 1 3. 21 x 10 -3 1. 10 x 10 -2 1. 30 x 10 -2 2 6. 40 x 10 -3 2. 20 x 10 -2 1. 30 x 10 -2 3 28. 8 x 10 -3 1. 10 x 10 -2 3. 90 x 10 -2 Rate law: rate = k[O 2]m[NO]n 16 -80 rate = k[O 2][NO]2
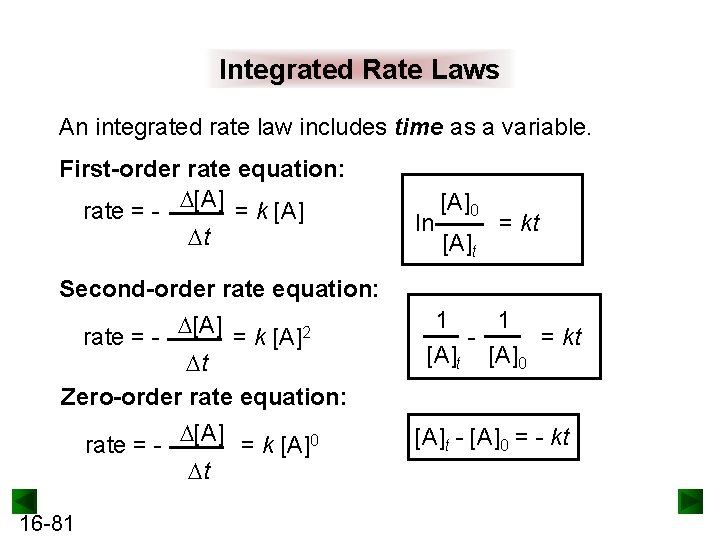
Integrated Rate Laws An integrated rate law includes time as a variable. First-order rate equation: rate = - [A] = k [A] t ln [A]0 [A]t = kt Second-order rate equation: rate = - [A] = k [A]2 t Zero-order rate equation: rate = - [A] = k [A]0 t 16 -81 1 1 = kt [A]0 [A]t - [A]0 = - kt
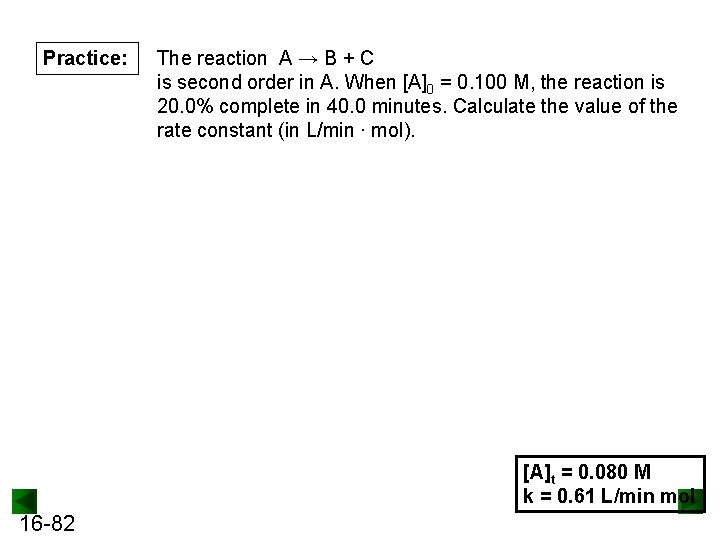
Practice: The reaction A → B + C is second order in A. When [A]0 = 0. 100 M, the reaction is 20. 0% complete in 40. 0 minutes. Calculate the value of the rate constant (in L/min ∙ mol). [A]t = 0. 080 M k = 0. 61 L/min mol 16 -82
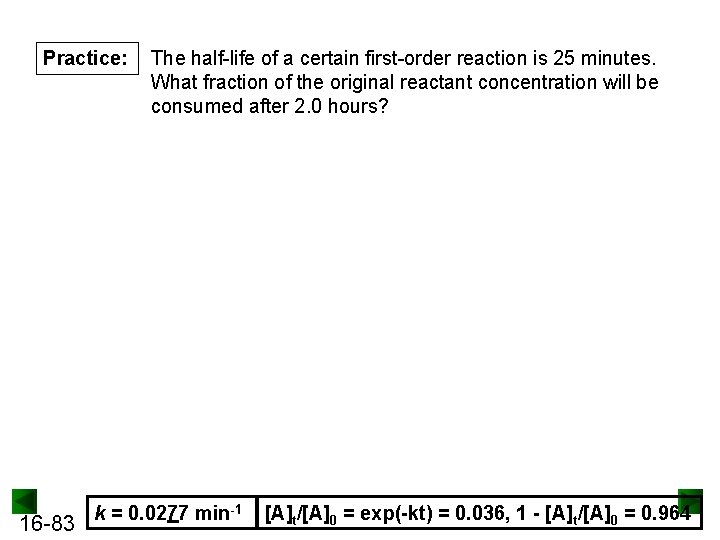
Practice: 16 -83 The half-life of a certain first-order reaction is 25 minutes. What fraction of the original reactant concentration will be consumed after 2. 0 hours? k = 0. 0277 min-1 [A]t/[A]0 = exp(-kt) = 0. 036, 1 - [A]t/[A]0 = 0. 964
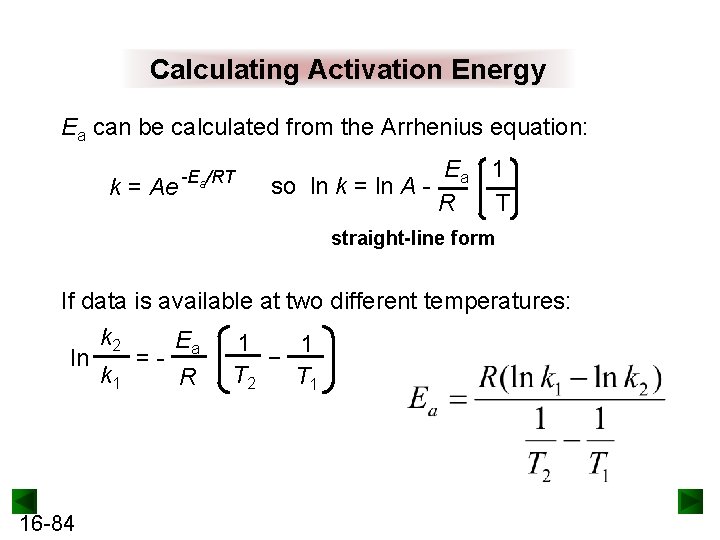
Calculating Activation Energy Ea can be calculated from the Arrhenius equation: k = Ae -Ea/RT Ea 1 so ln k = ln A R T straight-line form If data is available at two different temperatures: ln 16 -84 k 2 k 1 =- Ea R 1 1 − T 2 T 1
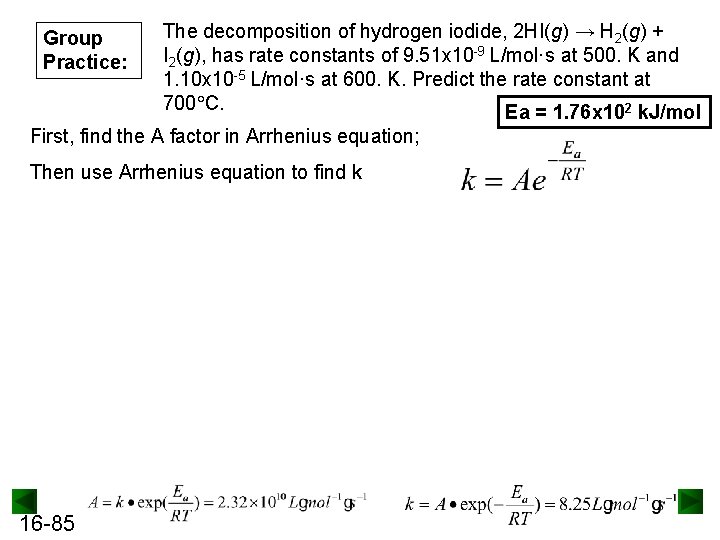
Group Practice: The decomposition of hydrogen iodide, 2 HI(g) → H 2(g) + I 2(g), has rate constants of 9. 51 x 10 -9 L/mol·s at 500. K and 1. 10 x 10 -5 L/mol·s at 600. K. Predict the rate constant at 700 C. Ea = 1. 76 x 102 k. J/mol First, find the A factor in Arrhenius equation; Then use Arrhenius equation to find k 16 -85
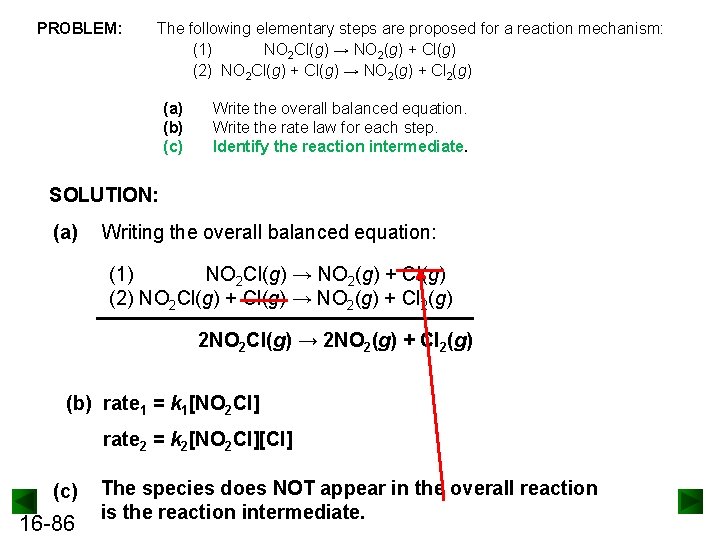
PROBLEM: The following elementary steps are proposed for a reaction mechanism: (1) NO 2 Cl(g) → NO 2(g) + Cl(g) (2) NO 2 Cl(g) + Cl(g) → NO 2(g) + Cl 2(g) (a) (b) (c) Write the overall balanced equation. Write the rate law for each step. Identify the reaction intermediate. SOLUTION: (a) Writing the overall balanced equation: (1) NO 2 Cl(g) → NO 2(g) + Cl(g) (2) NO 2 Cl(g) + Cl(g) → NO 2(g) + Cl 2(g) 2 NO 2 Cl(g) → 2 NO 2(g) + Cl 2(g) (b) rate 1 = k 1[NO 2 Cl] rate 2 = k 2[NO 2 Cl][Cl] (c) 16 -86 The species does NOT appear in the overall reaction is the reaction intermediate.
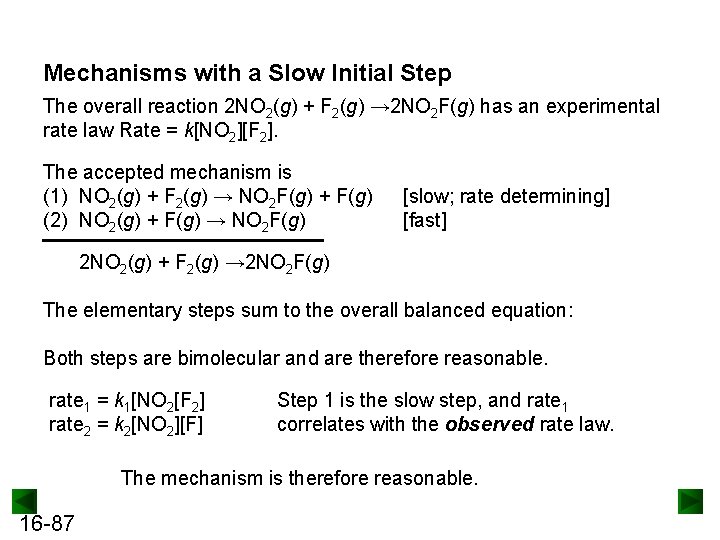
Mechanisms with a Slow Initial Step The overall reaction 2 NO 2(g) + F 2(g) → 2 NO 2 F(g) has an experimental rate law Rate = k[NO 2][F 2]. The accepted mechanism is (1) NO 2(g) + F 2(g) → NO 2 F(g) + F(g) (2) NO 2(g) + F(g) → NO 2 F(g) [slow; rate determining] [fast] 2 NO 2(g) + F 2(g) → 2 NO 2 F(g) The elementary steps sum to the overall balanced equation: Both steps are bimolecular and are therefore reasonable. rate 1 = k 1[NO 2[F 2] rate 2 = k 2[NO 2][F] Step 1 is the slow step, and rate 1 correlates with the observed rate law. The mechanism is therefore reasonable. 16 -87
Section 2 reinforcement classifying chemical reactions
Section 2 classifying chemical reactions worksheet answers
Chemical reactions section 3 reactions in aqueous solutions
Unit ratio
Ratios guided notes
Ratios rates and unit rates
Ratios rates and unit rates
Section 1 chemical changes
Chapter 18 chemical reactions balancing chemical equations
Molecularity of reaction
Grade 11 chemistry unit 4
Half life chemistry kinetics
Chemical kinetics definition
Chemical kinetics experiment
Applications of chemical kinetics
Steady state approximation examples
An example of redox reaction
Chemistry unit 5 reactions balancing reactions worksheet
Chemical reactions reactants and products
Chemistry chapter 8 review chemical equations and reactions
Chapter 8 section 1 chemical equations and reactions
Chapter 8 review chemical equations and reactions
Section 2-4 chemical reactions and enzymes
Unit 5 chemical reactions
Types of chemical reactions and solution stoichiometry
Unit 5 chemical equations and reactions
Types of chemical reactions and solution stoichiometry
Percent yield of copper lab
What is released or absorbed whenever chemical
Section 2-4 chemical reactions and enzymes
Building vocabulary: chemical bonds and reactions
Kinetics and equilibrium
Explain law of mass action
Zero order kinetics
Planar kinetics of a rigid body: force and acceleration
Kinetics of a particle: force and acceleration
Kinetics of a particle: impulse and momentum
Promonocyte
Kinematic of rigid body
Planar kinetics of a rigid body work and energy
Fermenter
Proportional relationships in chemical reactions
Different types of redox reactions
Types of reaction
Types of reactions chemistry
Types of reactions
Predicting products of chemical reactions
4 types of chemical reactions
Non examples of chemical reactions
Chapter 10 chemical reactions answer key
The calculation of quantities in chemical reactions
Ouchterlony
Predicting products of chemical reactions
Chemistry predicting products
Combination reaction equation
Unit 11 chemical reactions
Lesson 68 toxic reactions chemical equations
Four types of chemical reactions
Biology-roots.com
Describing chemical reactions
Chemical reactions classification
Examples of chemical reactions in everyday life
What are the five chemical changes
5 general types of chemical reactions
Chemical reactions study guide
Chapter 9 chapter assessment chemical reactions
Equilibrium of chemical reactions
Chapter 9 chemical reactions
What are the 4 types of chemical reactions
Four types of chemical reactions
Chapter 9 study guide chemical reactions
5 chemical reactions
What are the 5 types of chemical reactions
Chapter 11 chemical reactions answer key
Predict the products of the following reactions.
Functional isomers of carboxylic acids
Chemical reactions summary
Yeast chemical reaction
Solvent in chemical reactions
Fatoumata dembele chef
Mass relationships in chemical reactions
Indications of chemical reactions
Describing chemical reactions
Rules of chemical reaction
Rearranging chemical equations
Chapter 19 chemical reactions simple word equations
Classification of chemical reactions worksheet
Combustion chemical reaction