MODERN PHYSICS Department of Physics Faculty of Science
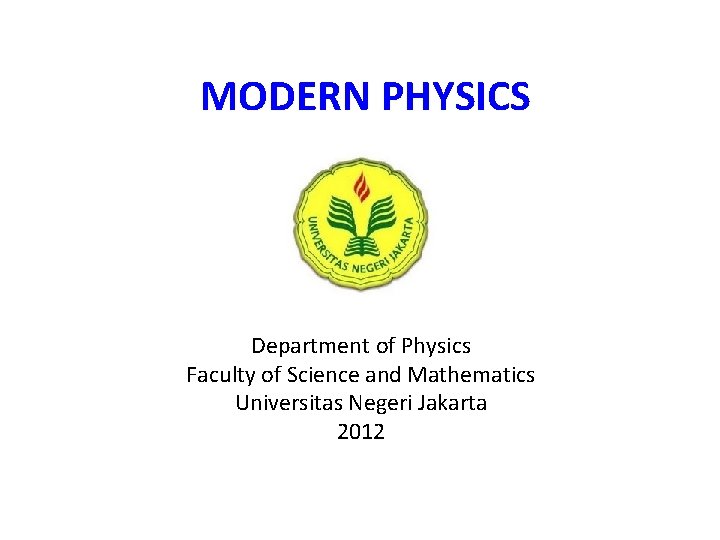
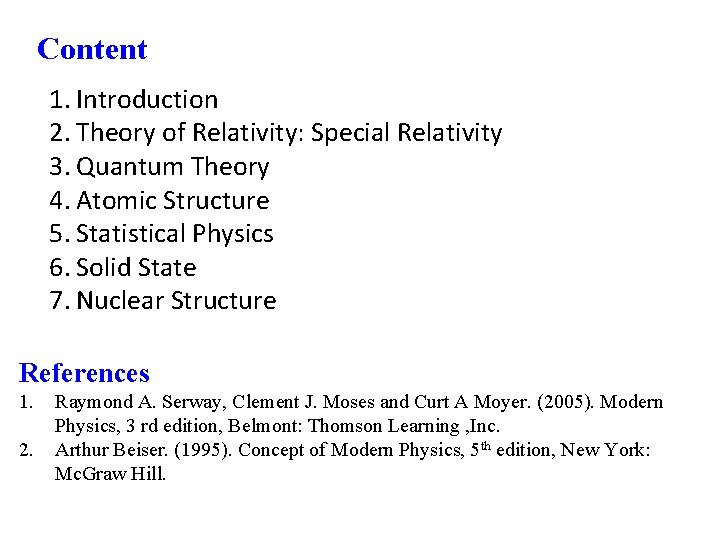
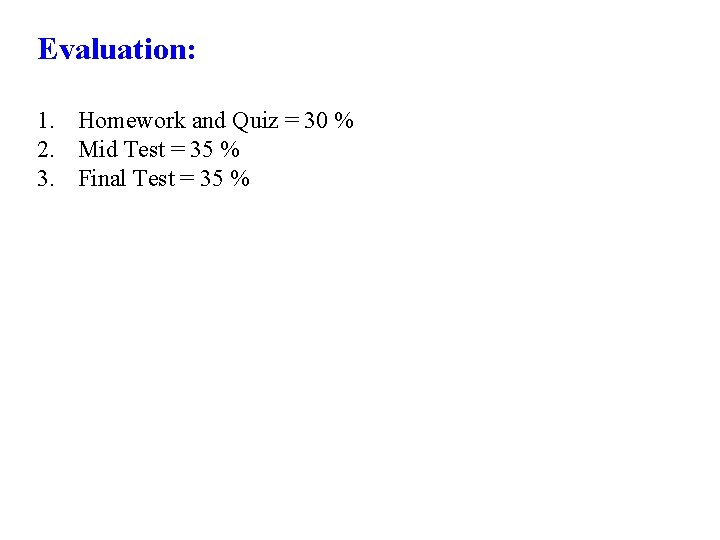
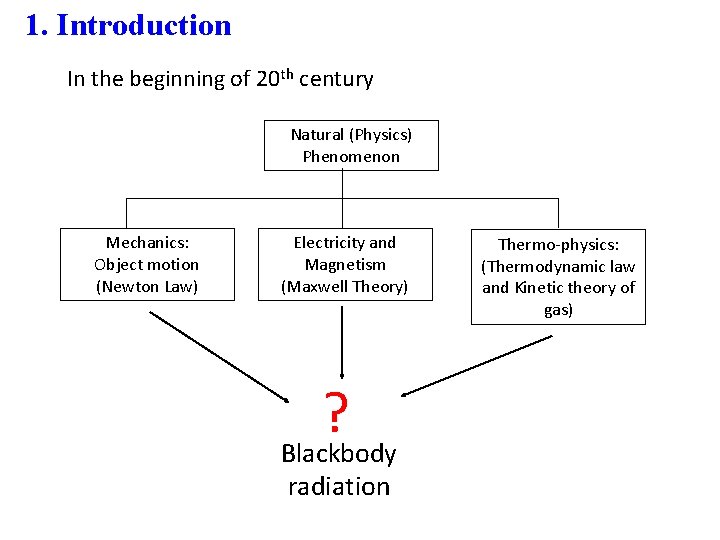
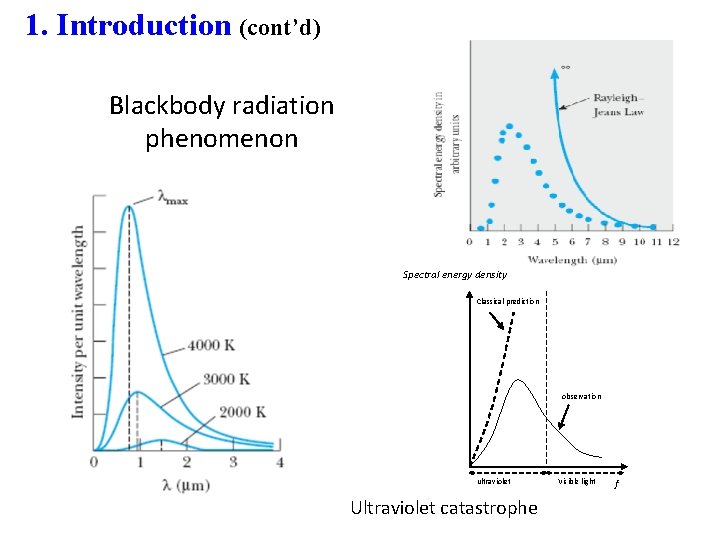
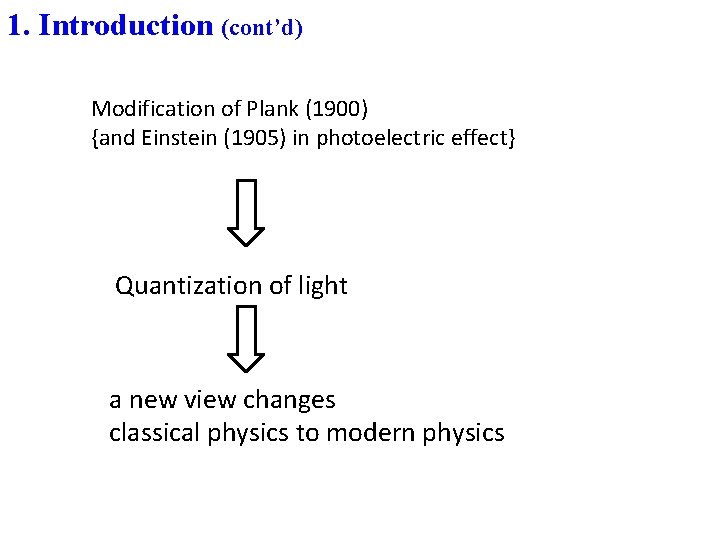
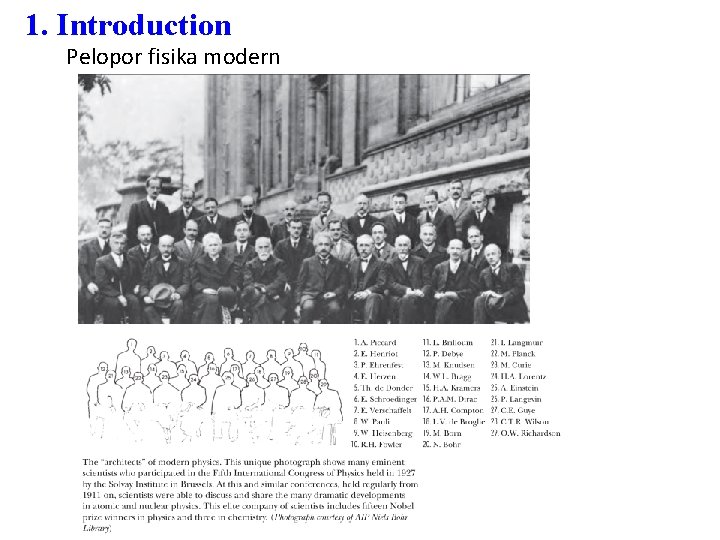
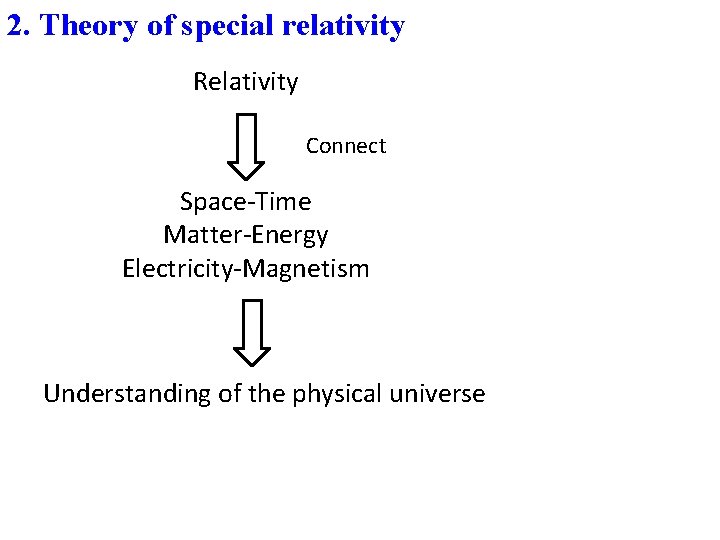
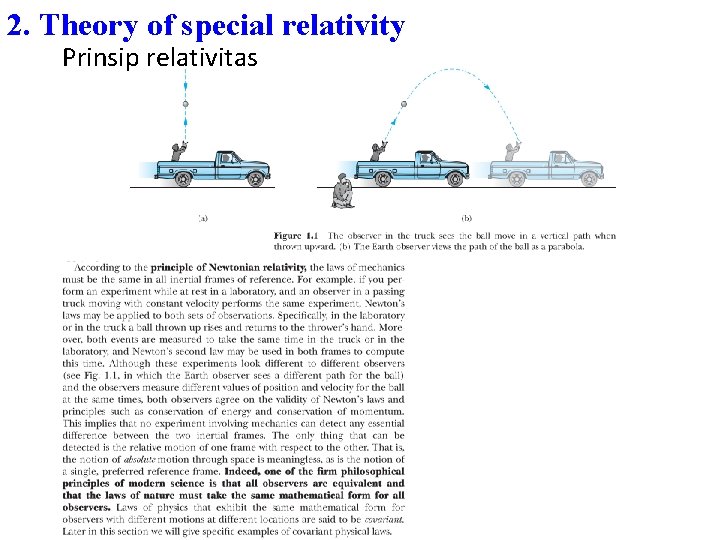
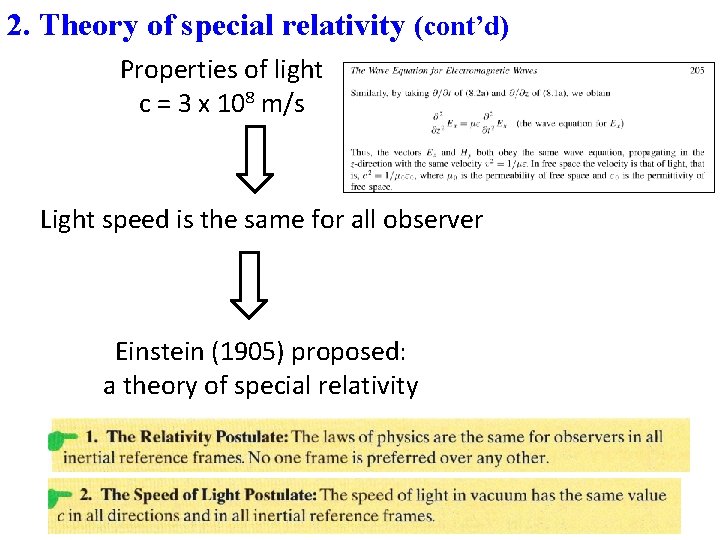
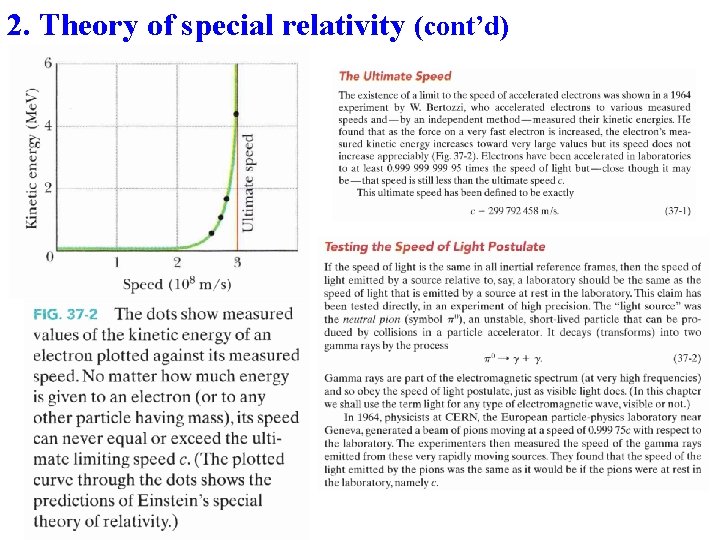
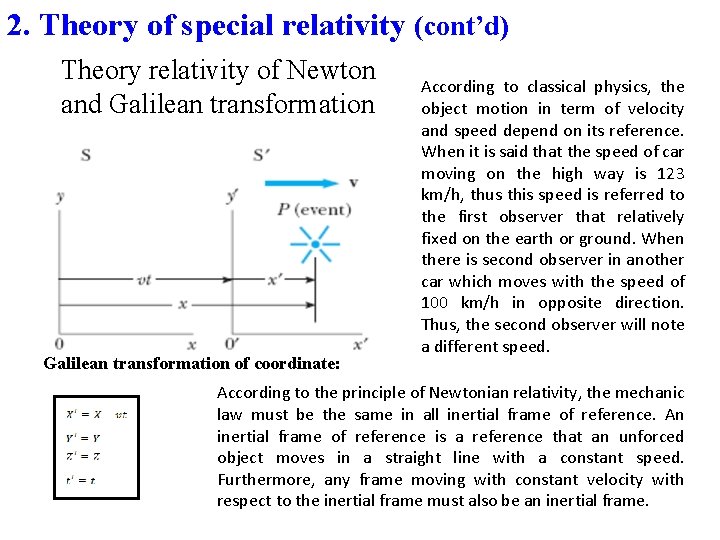
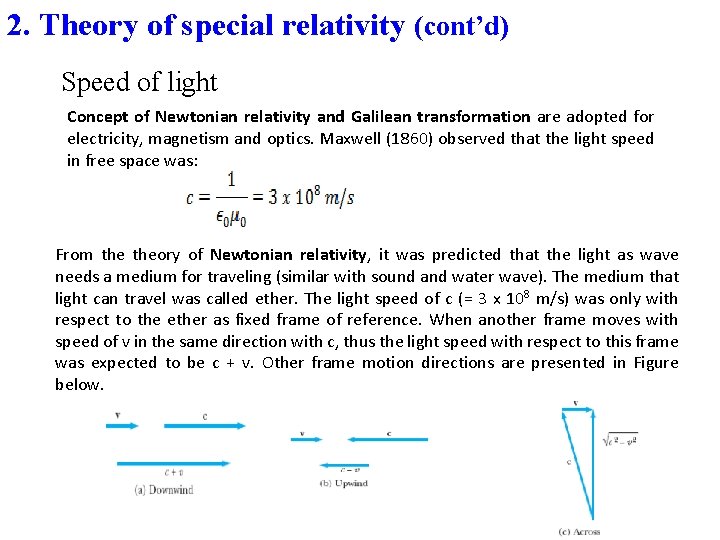
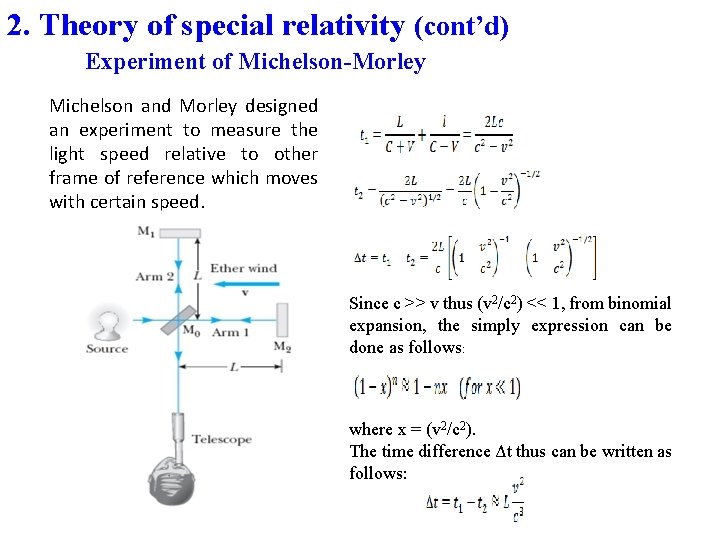
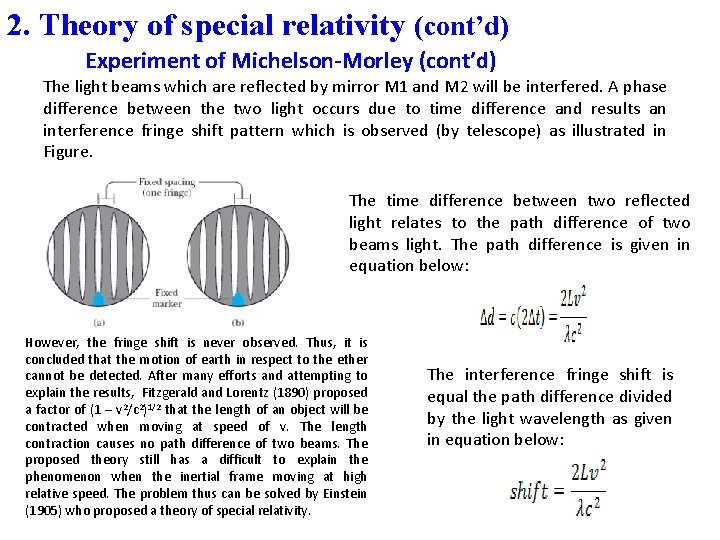
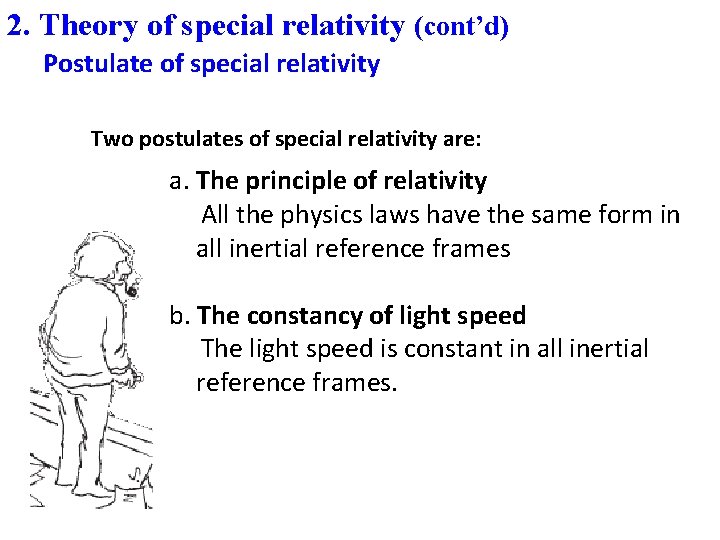
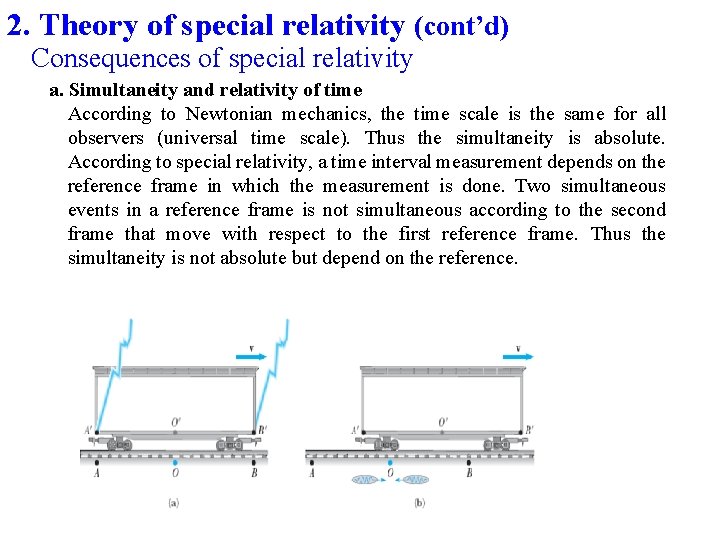
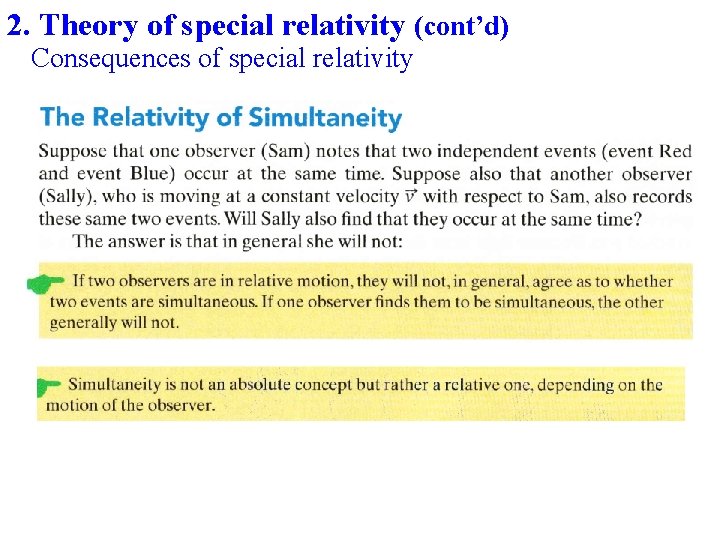
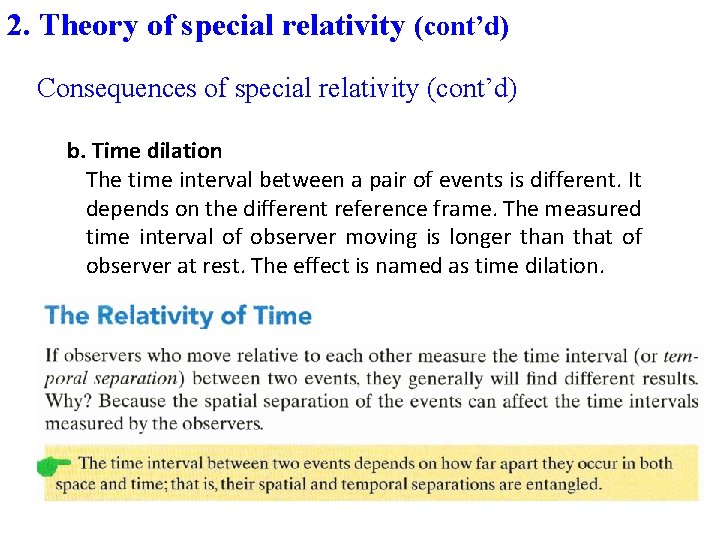
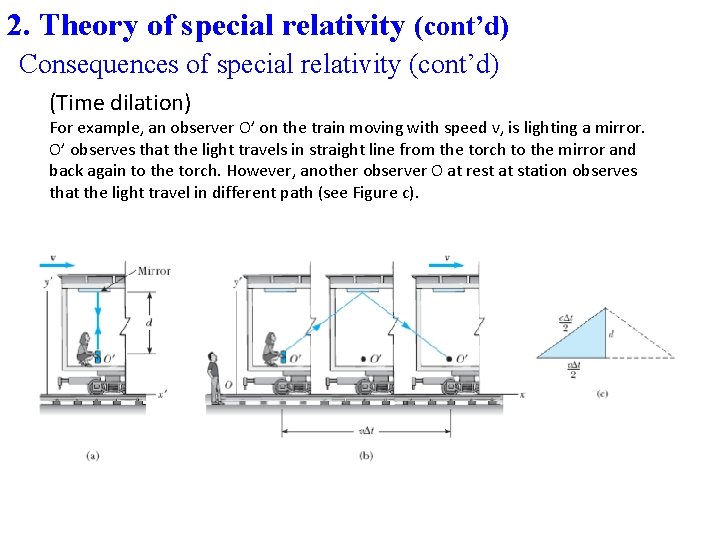
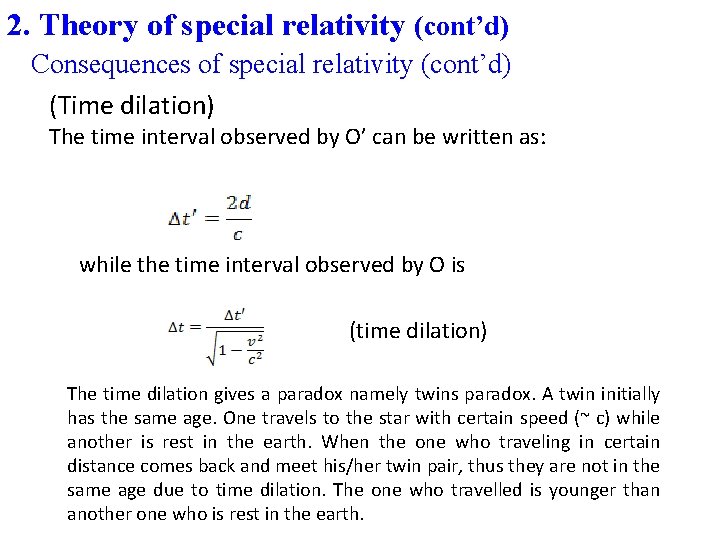
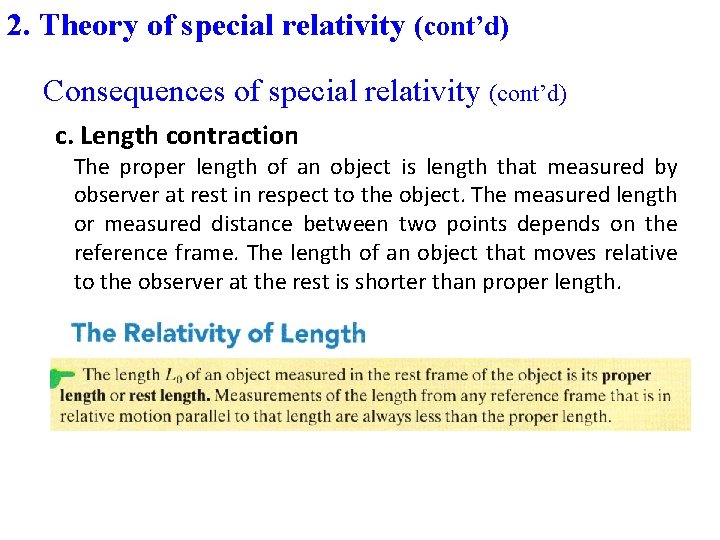
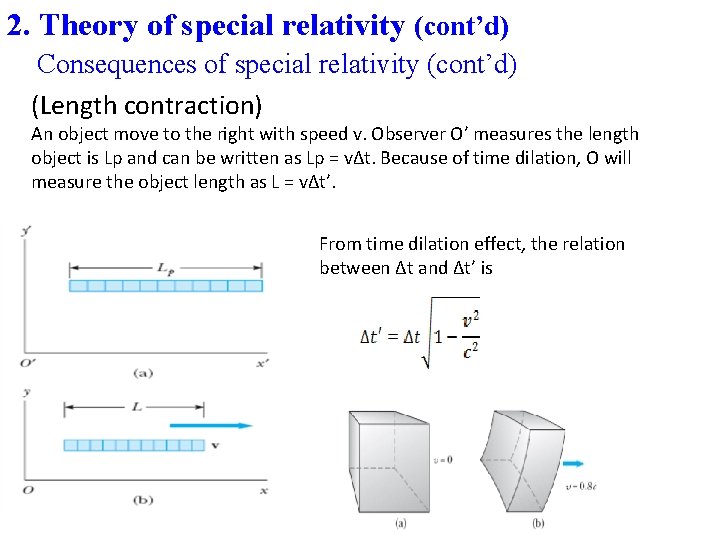
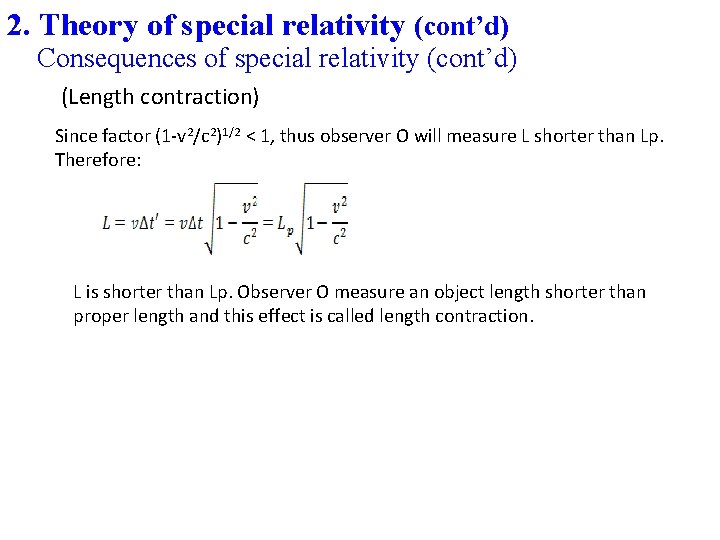
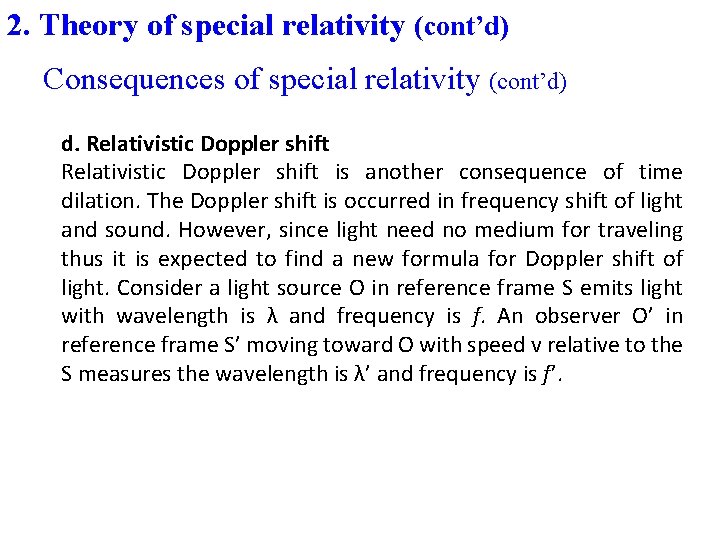
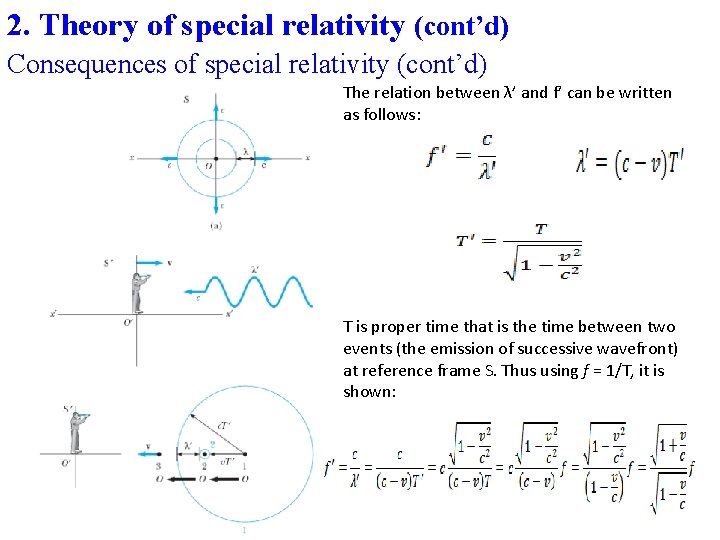
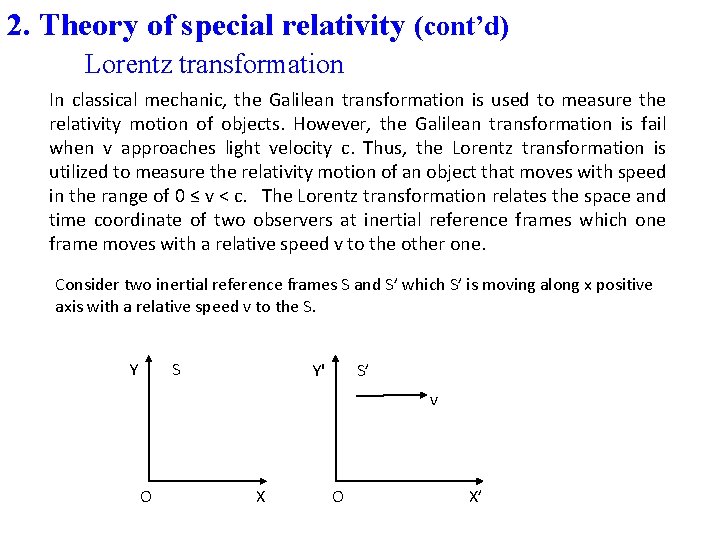
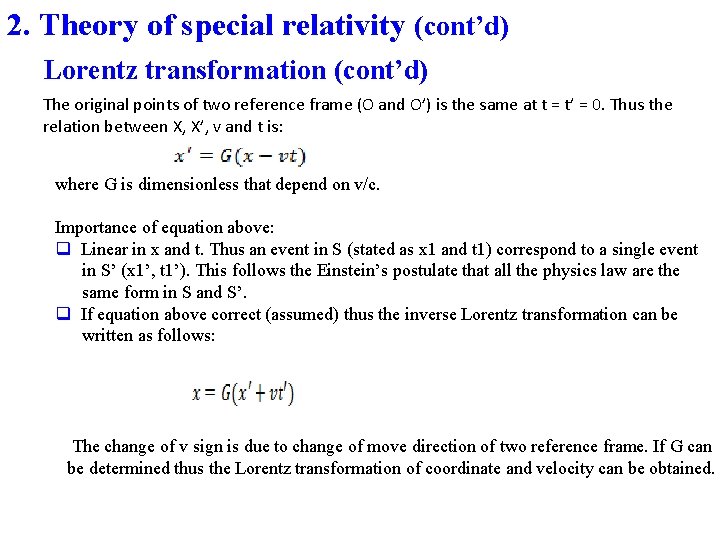
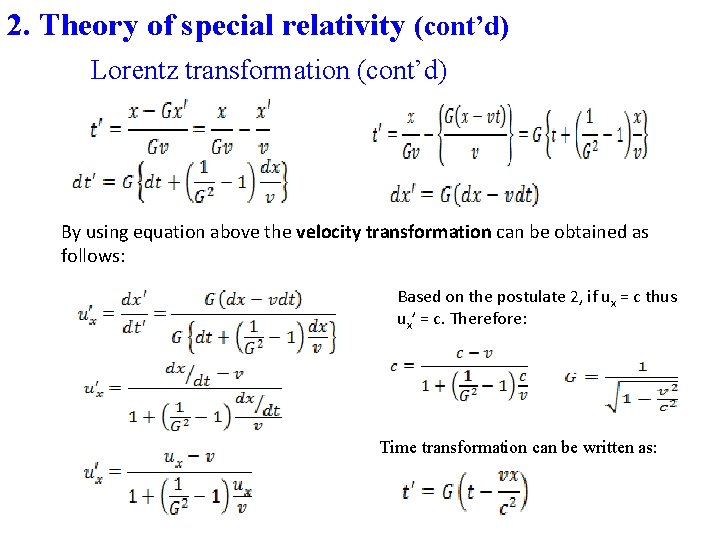
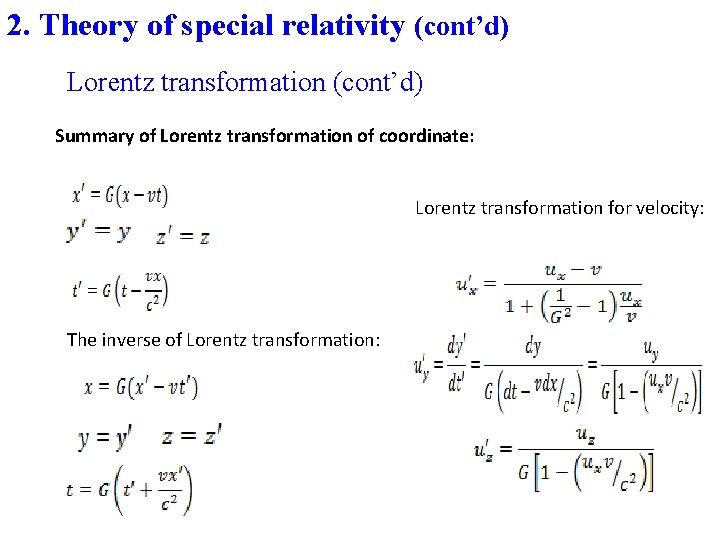
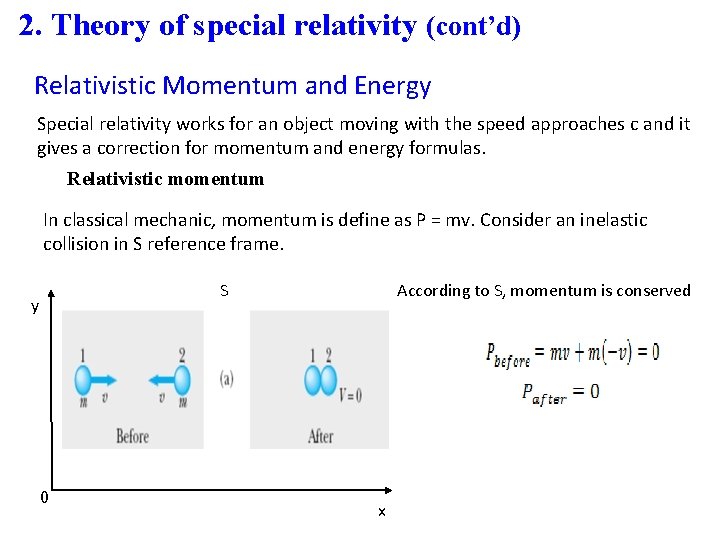
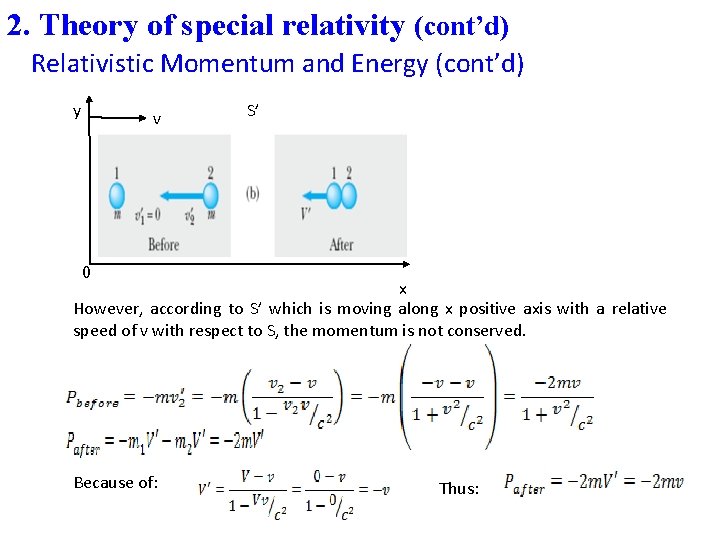
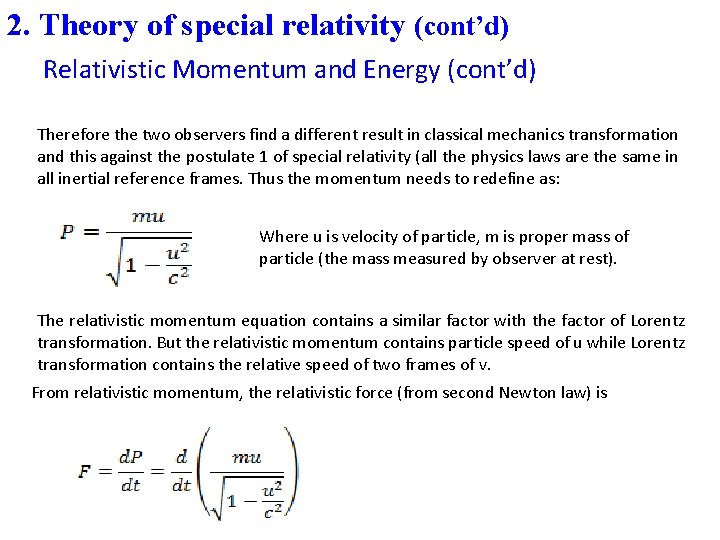
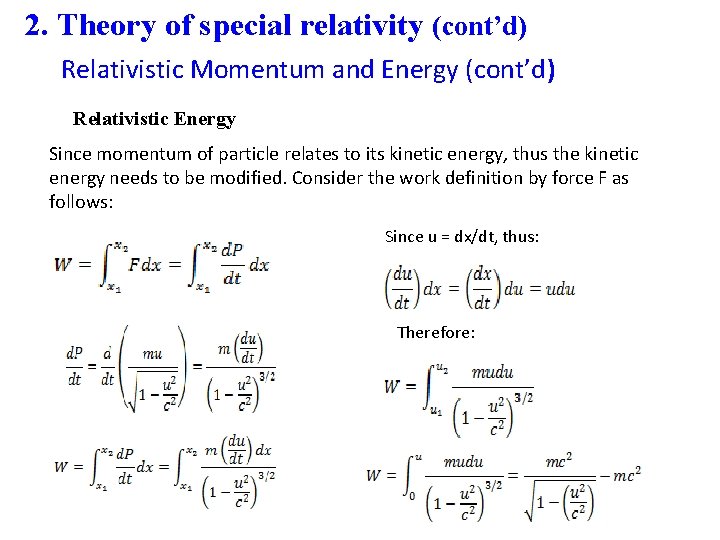
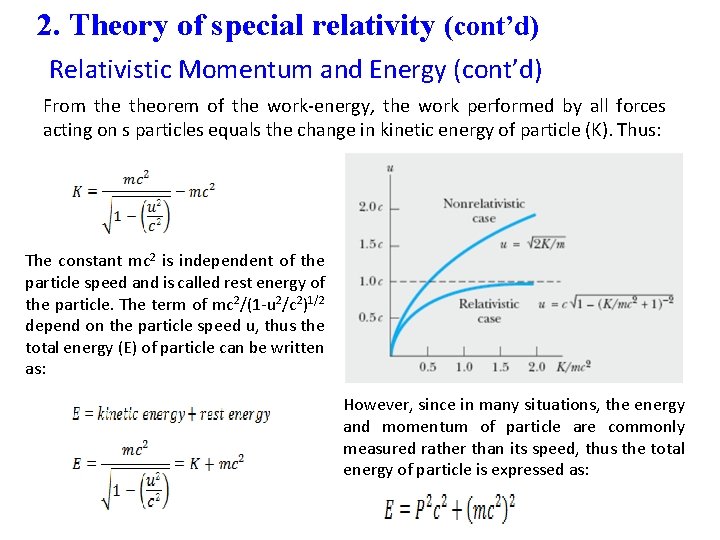
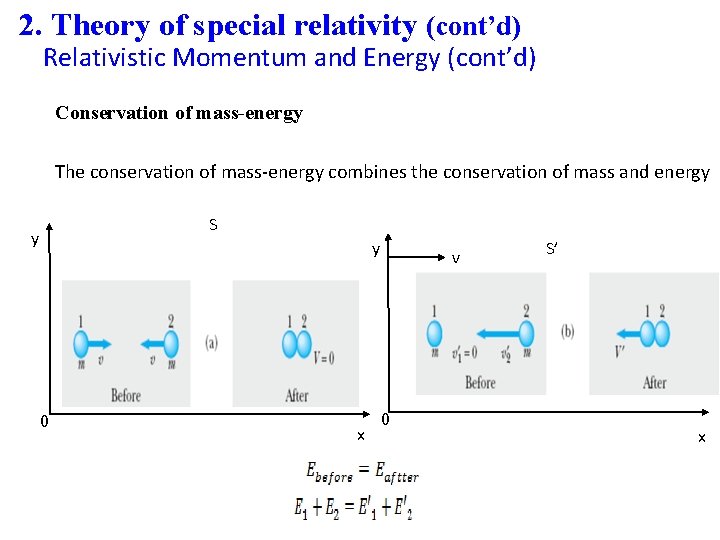
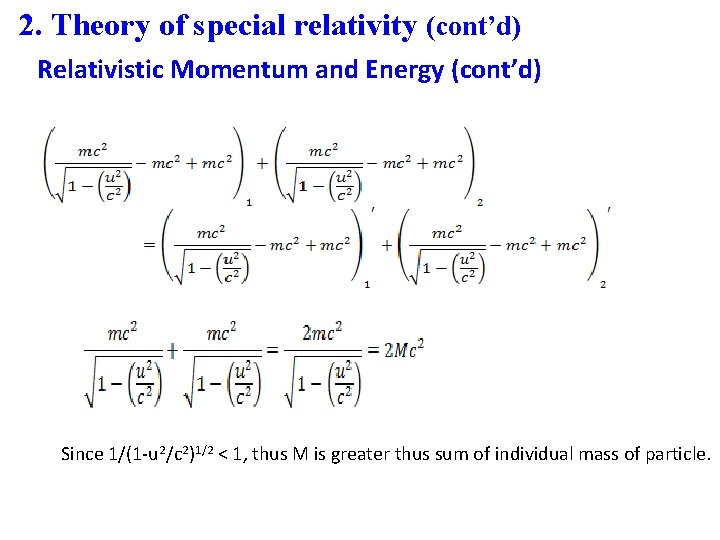
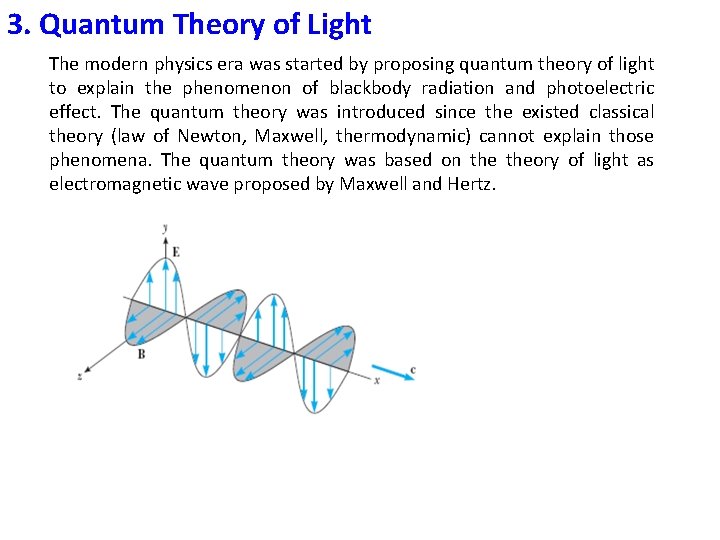
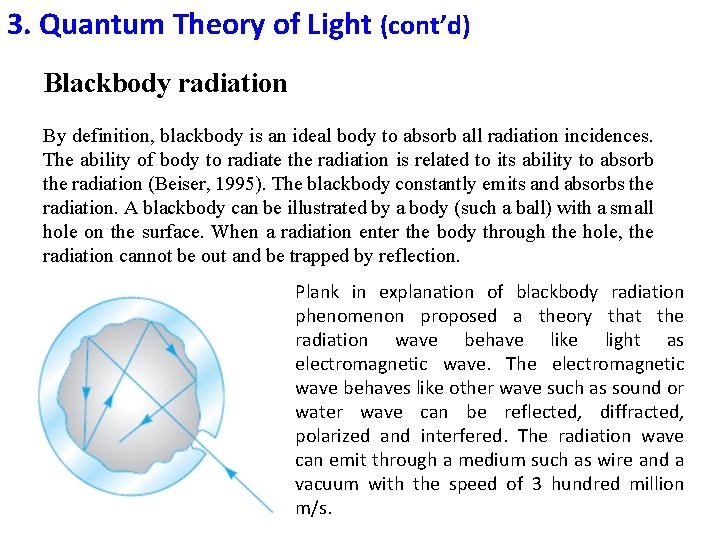
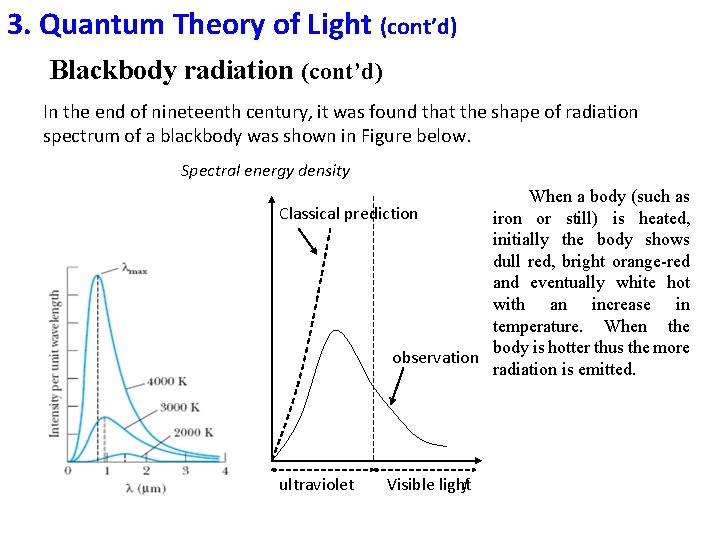
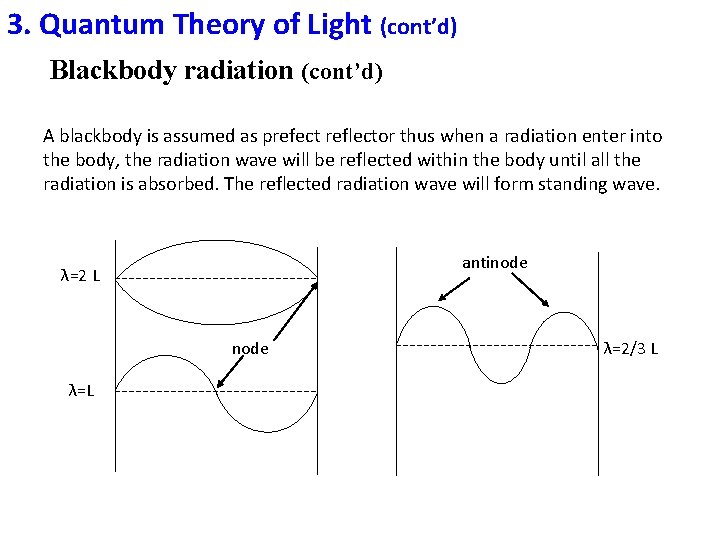
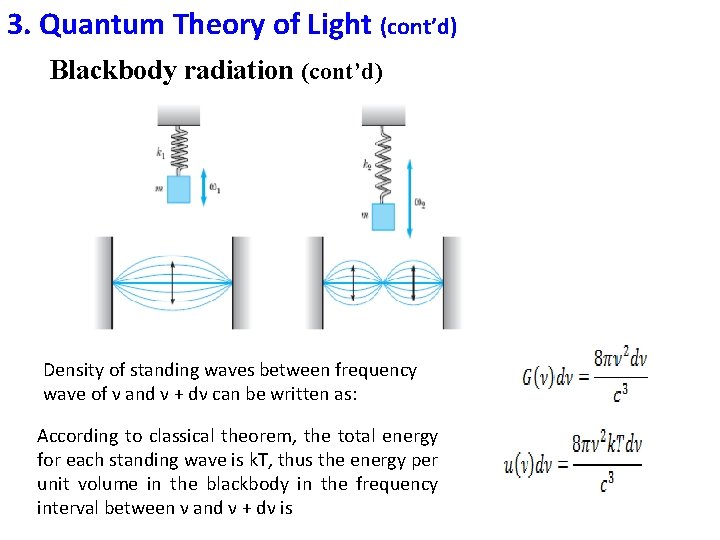
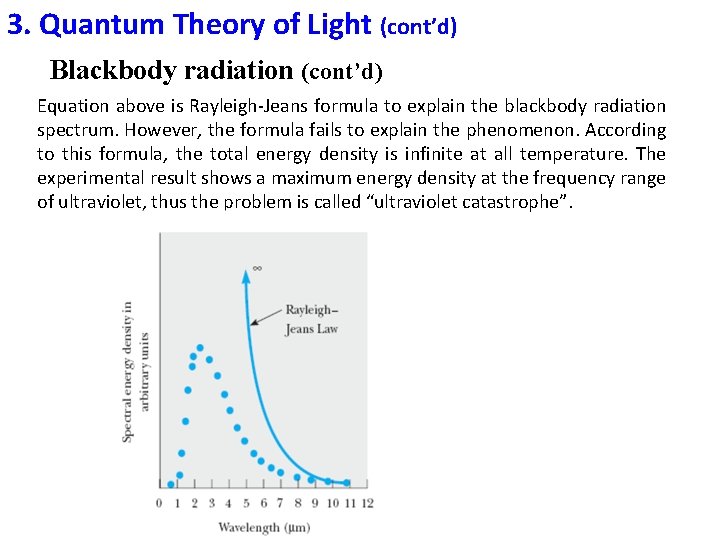
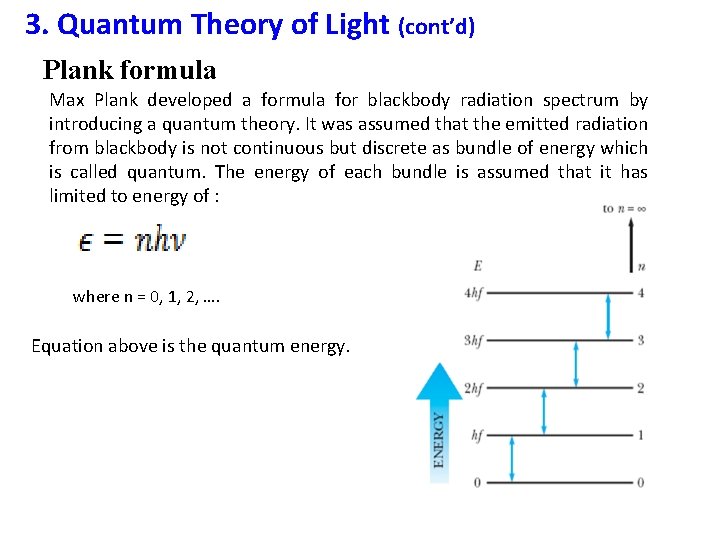
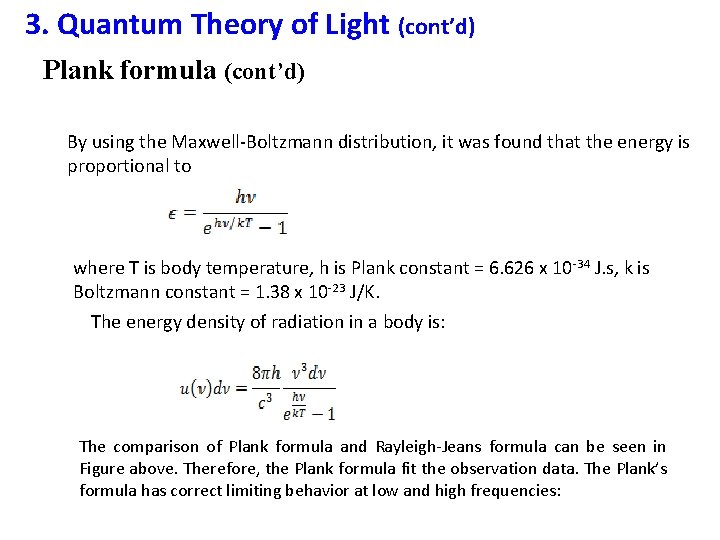
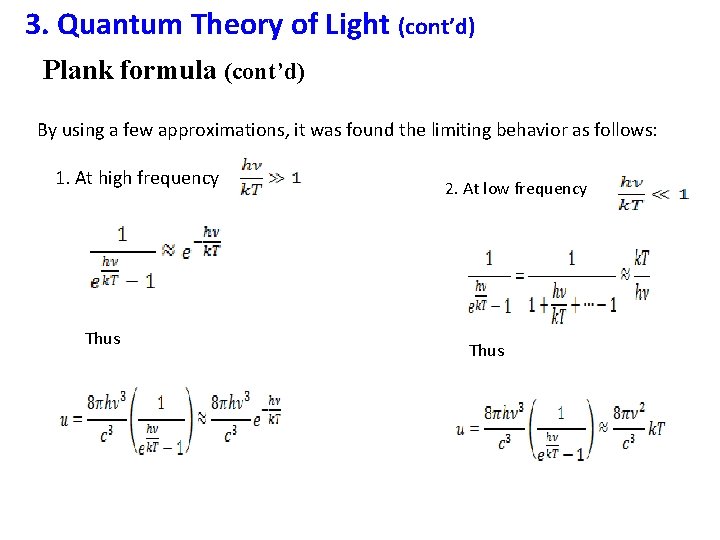
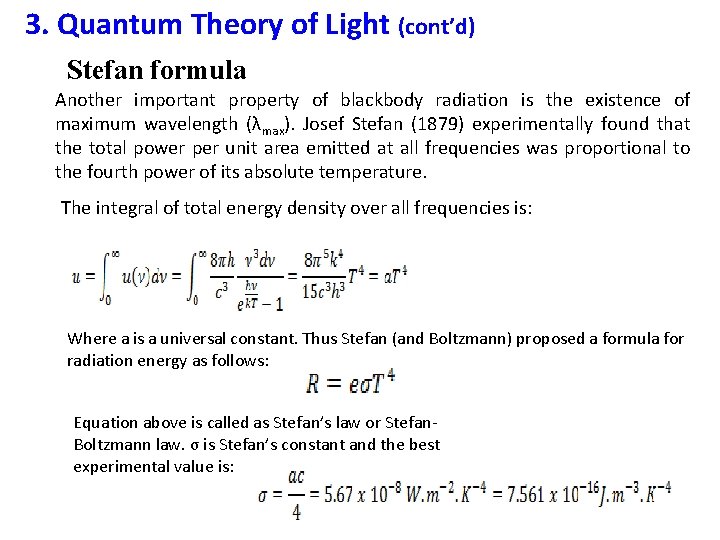
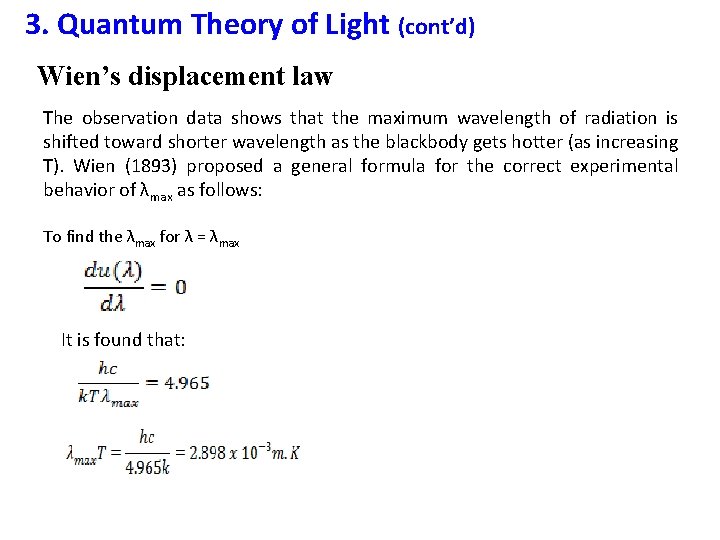
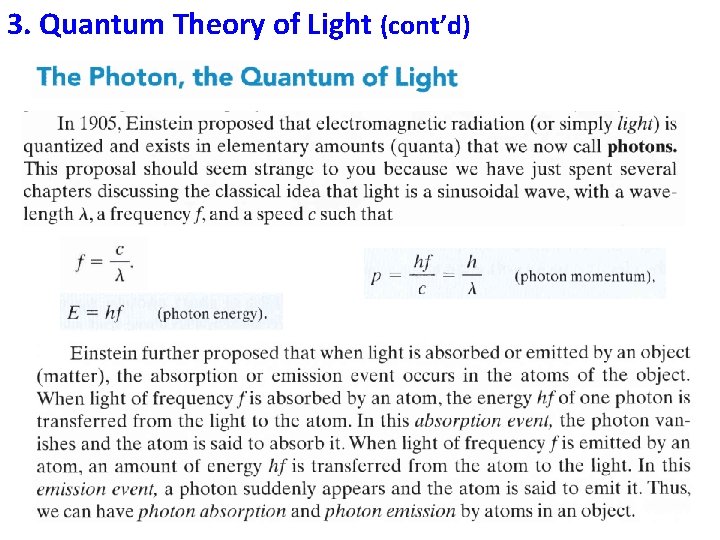
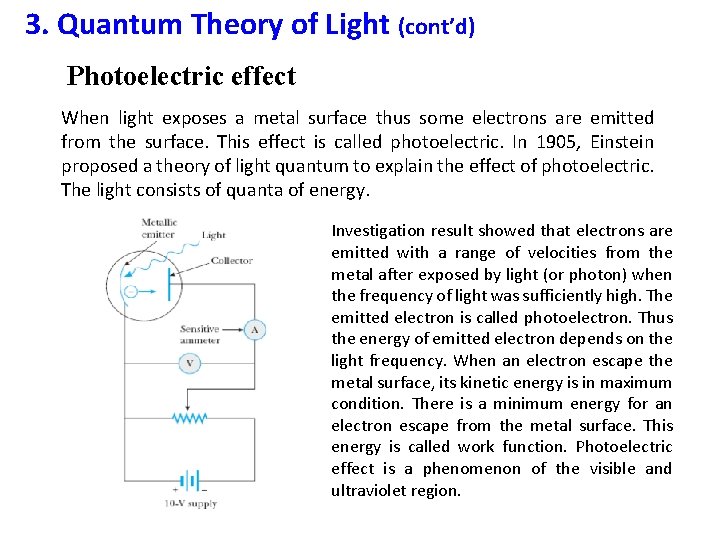
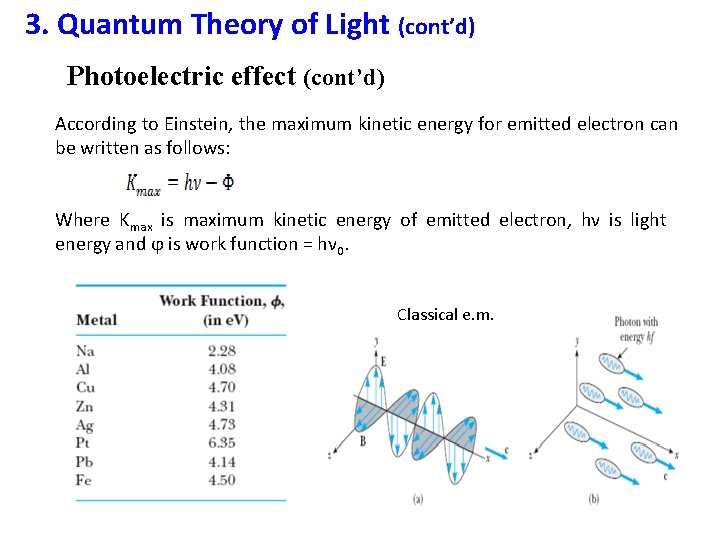
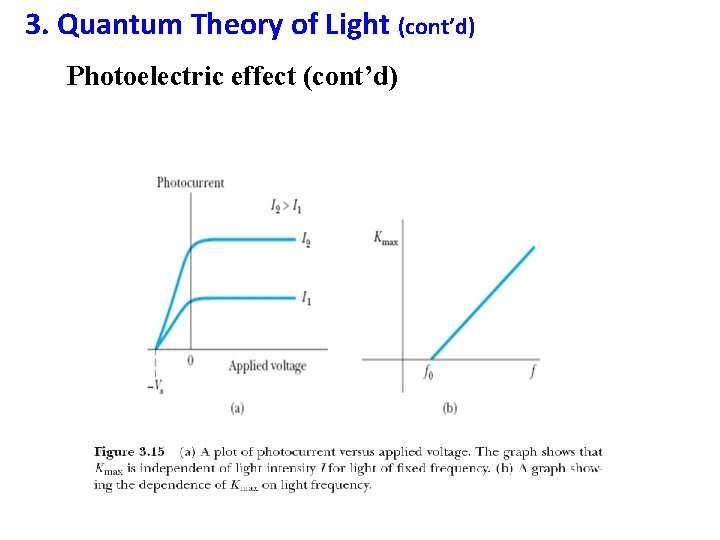
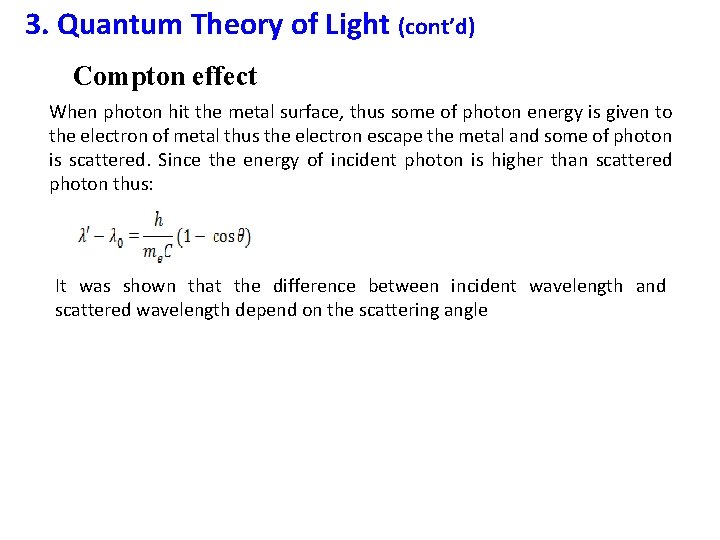
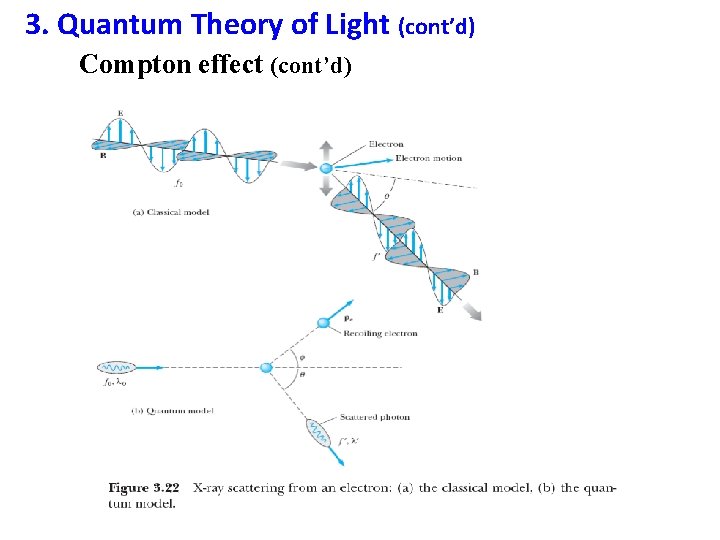
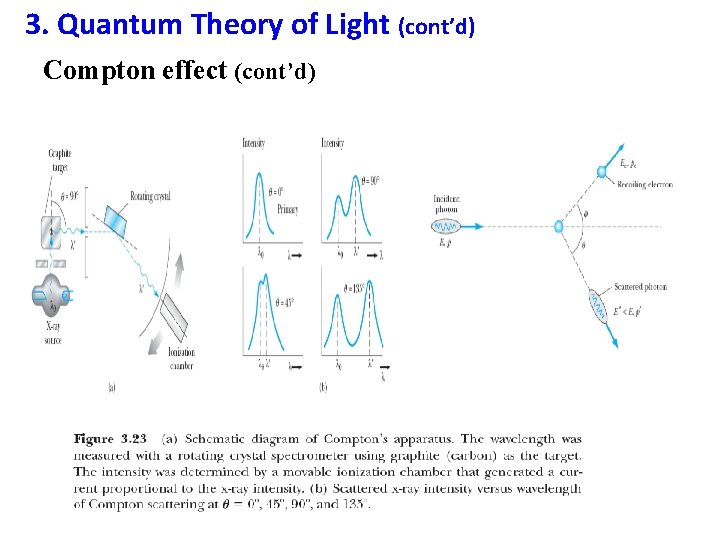
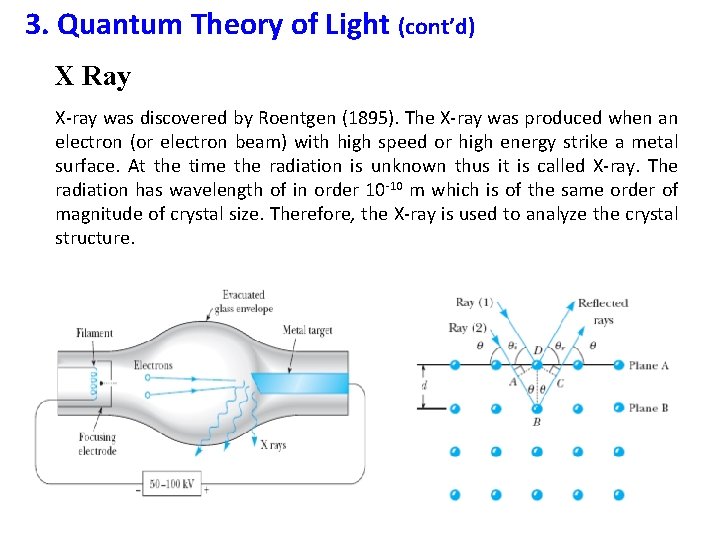
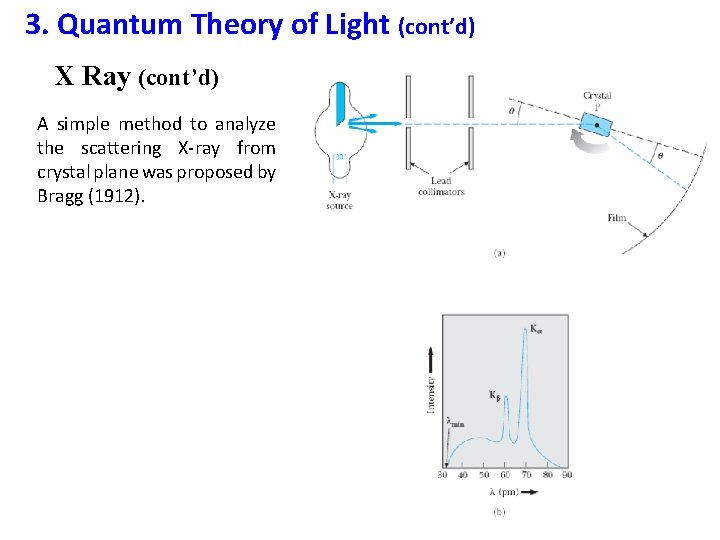
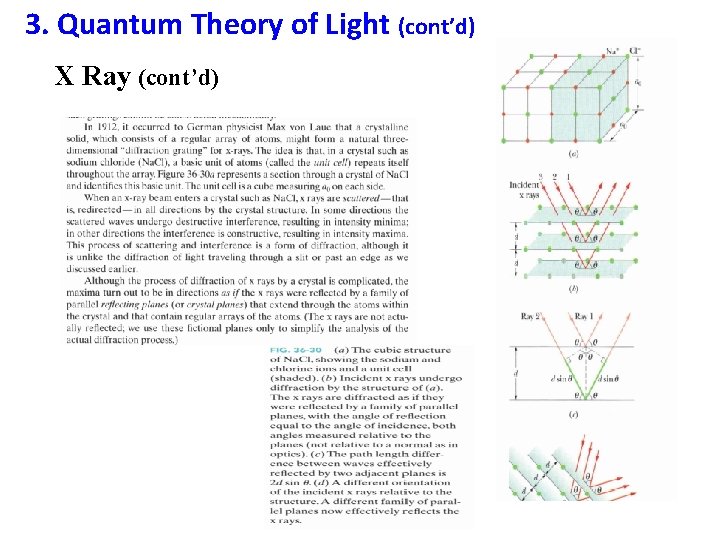
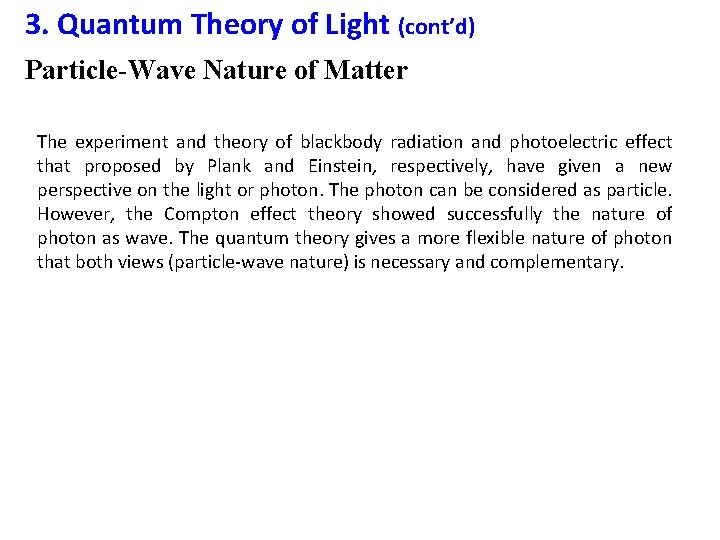
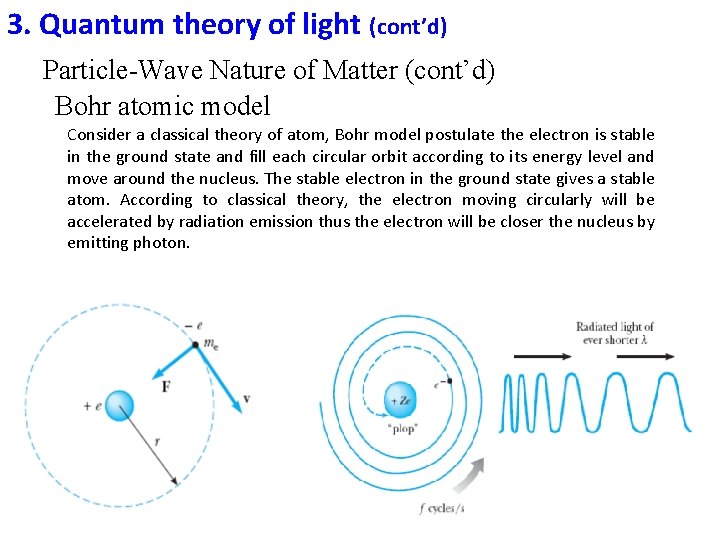
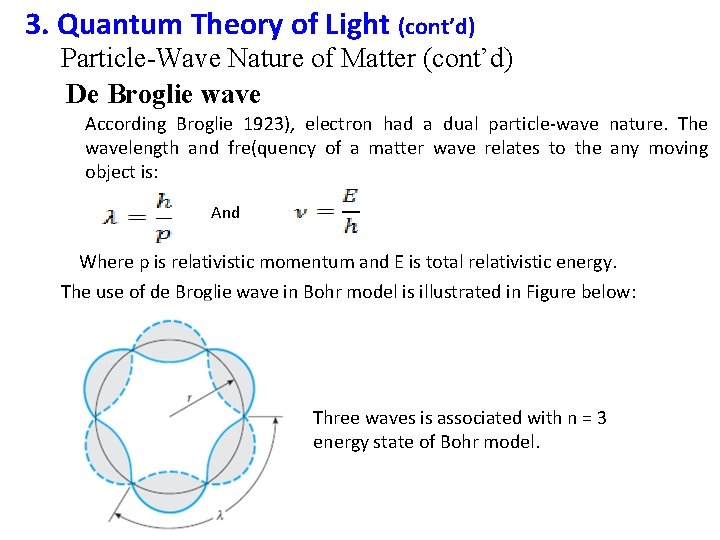
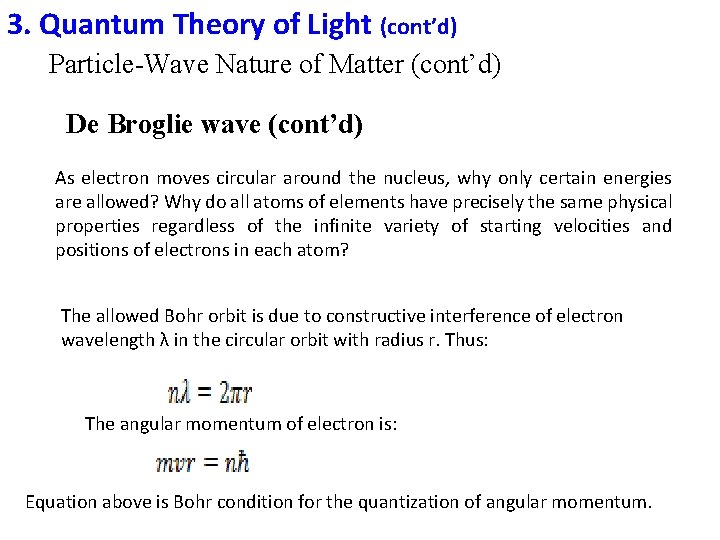
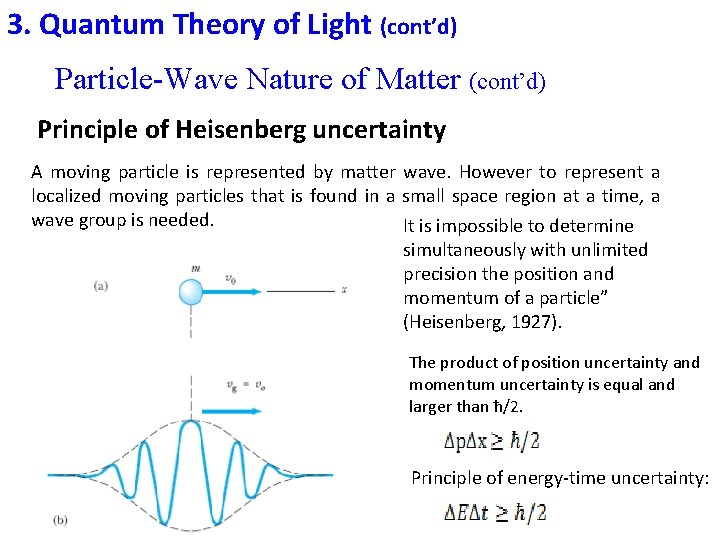
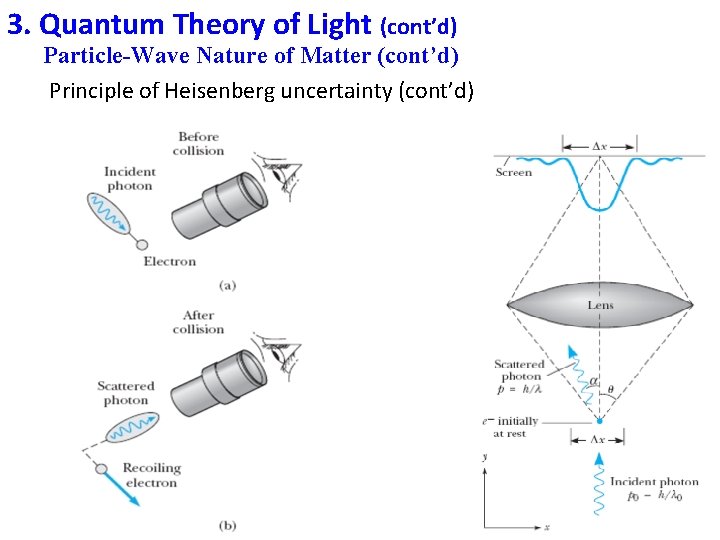
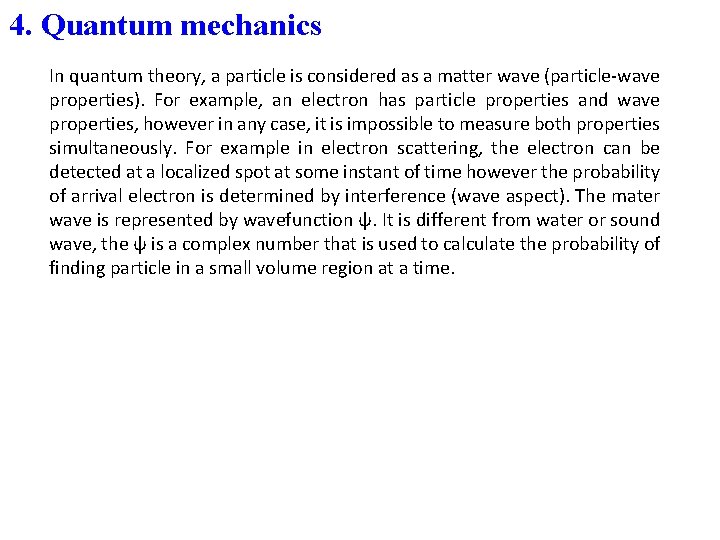
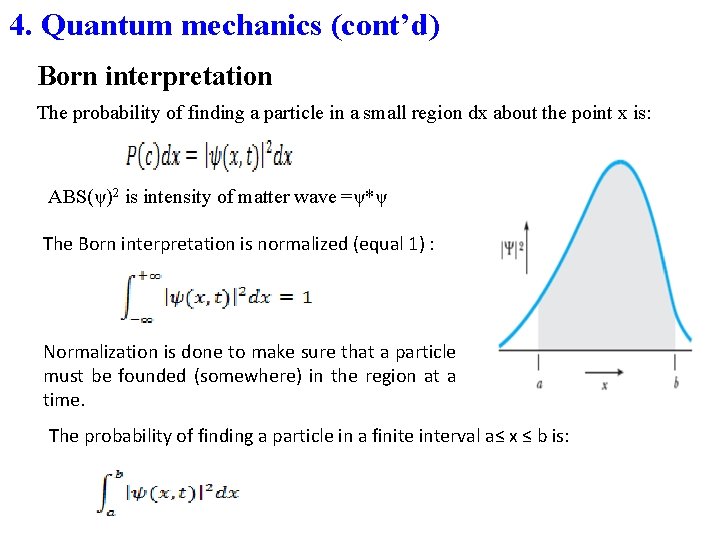
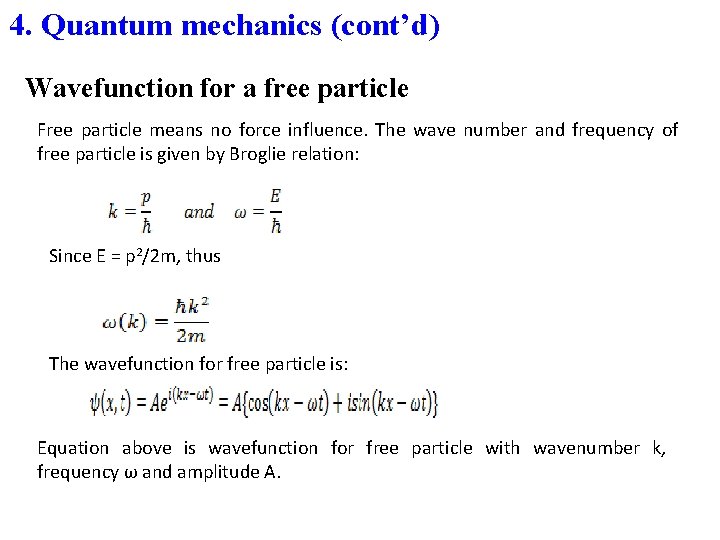
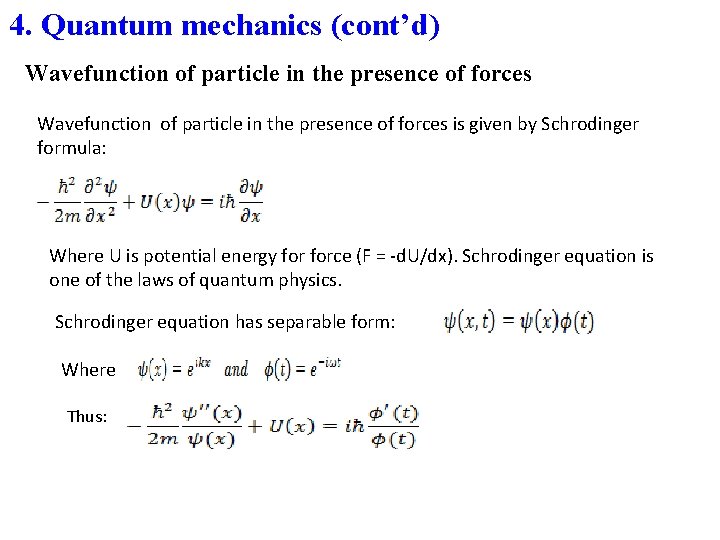
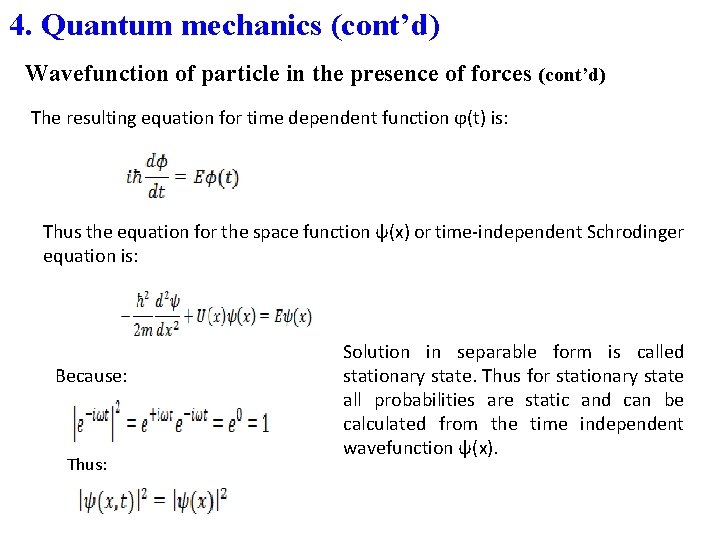
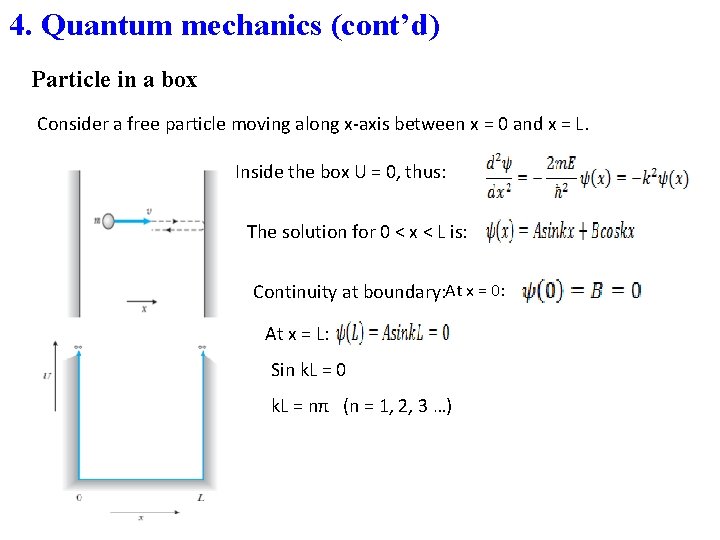
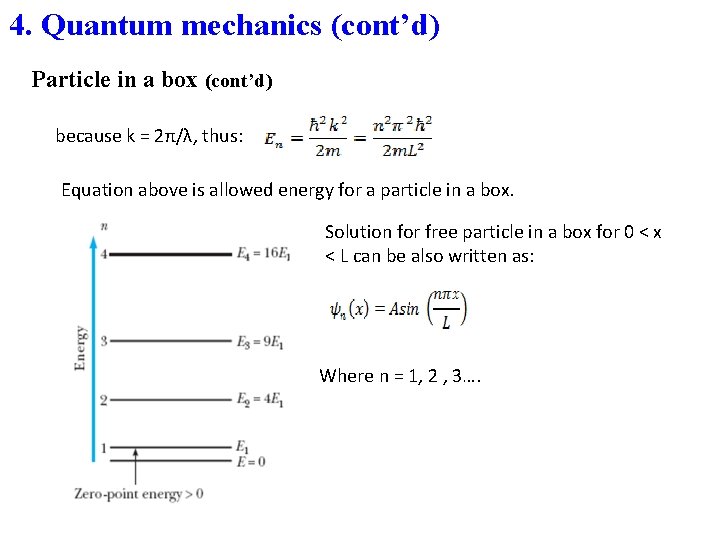
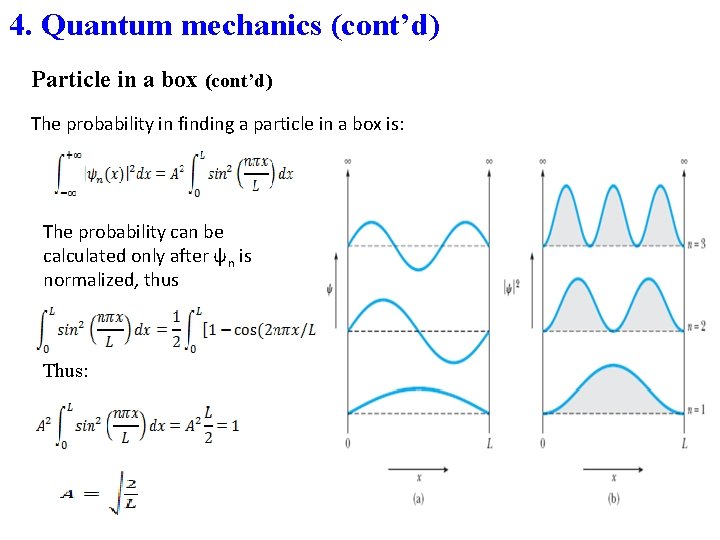
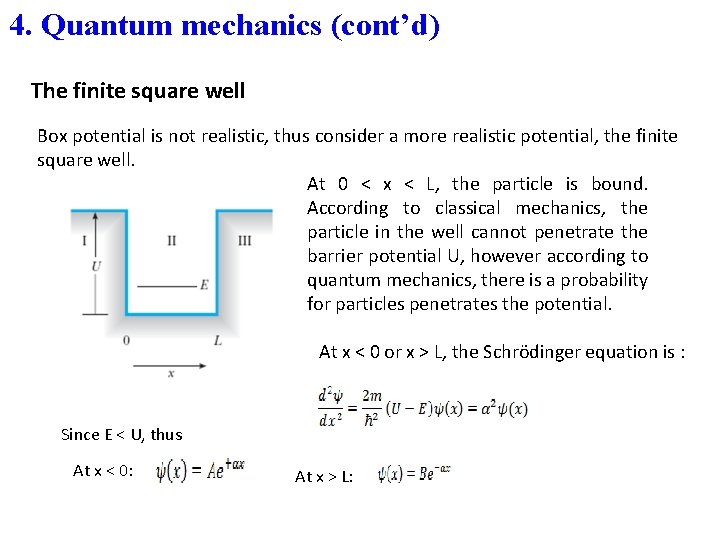
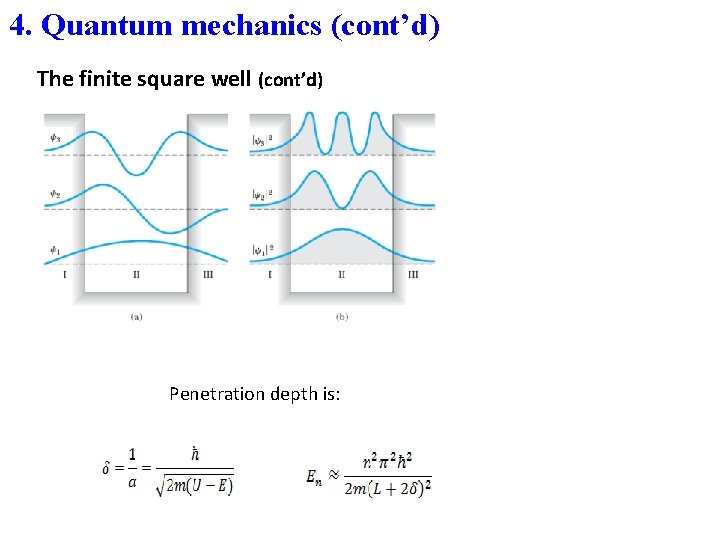
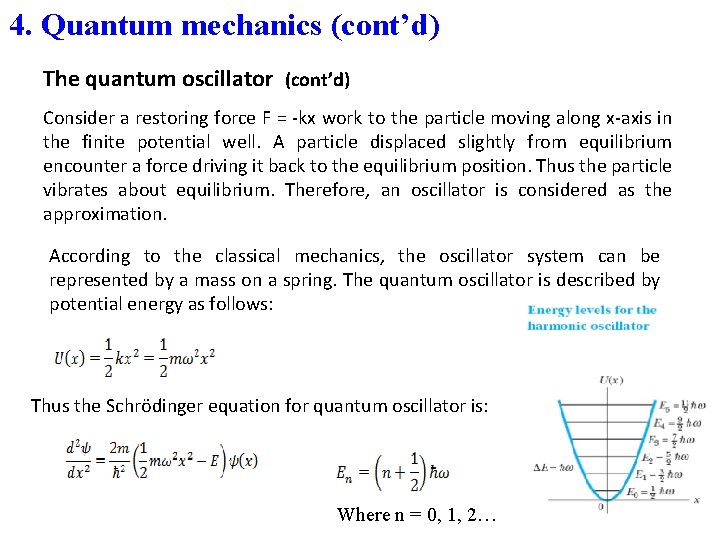
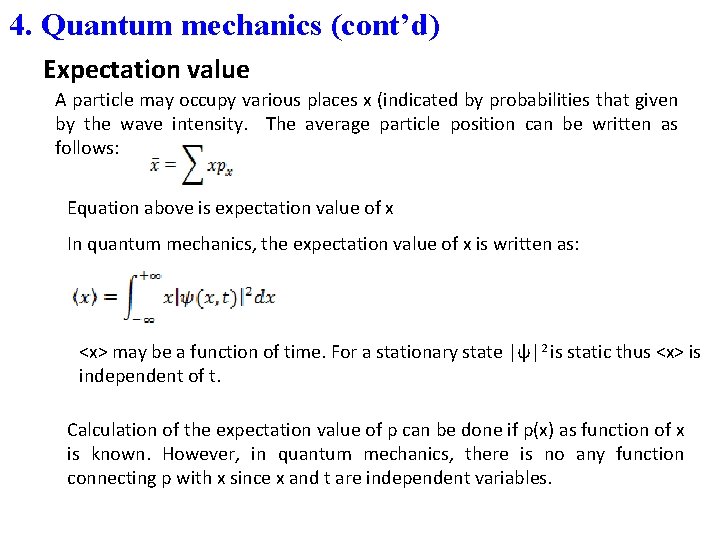
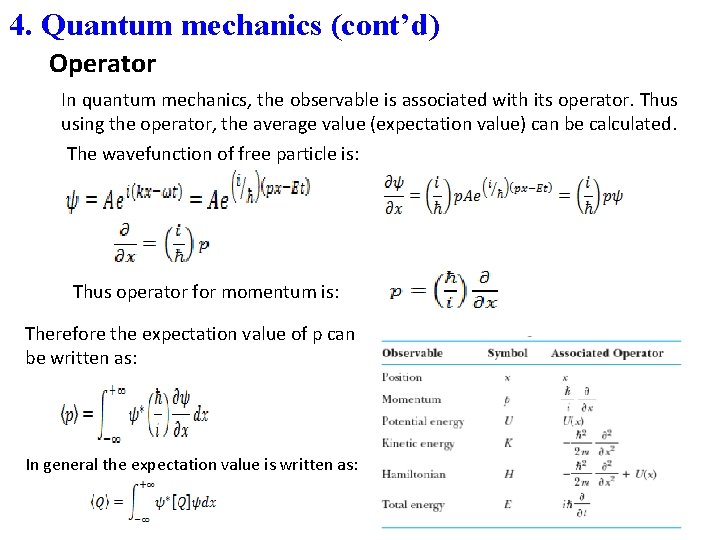
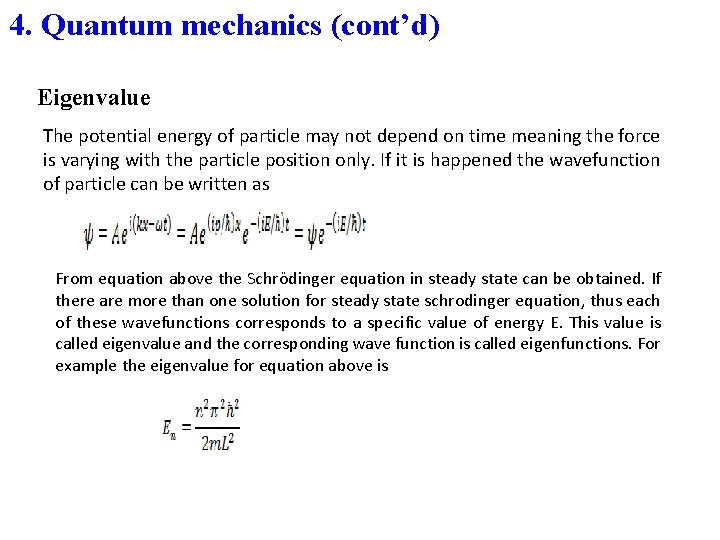
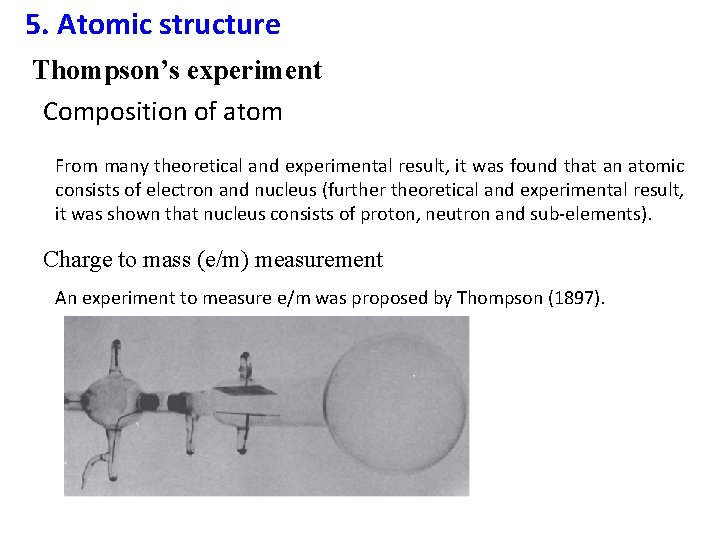
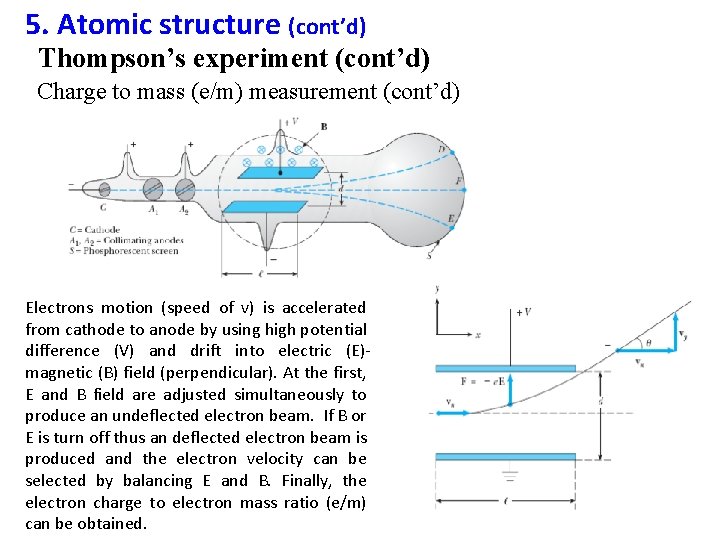
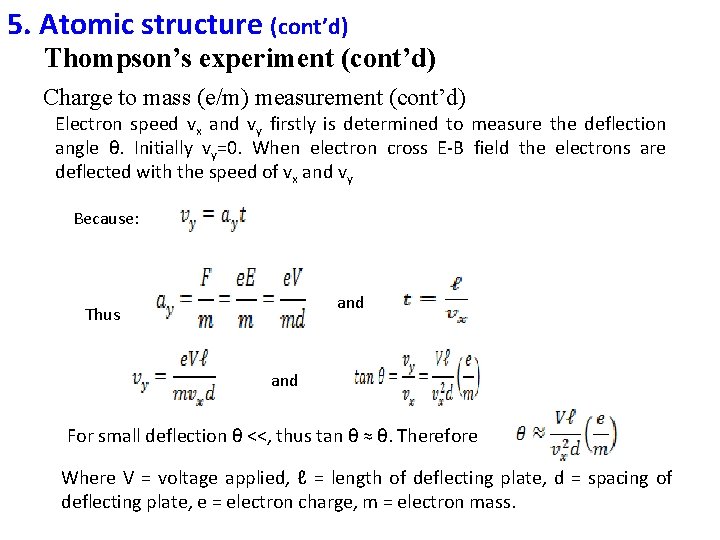
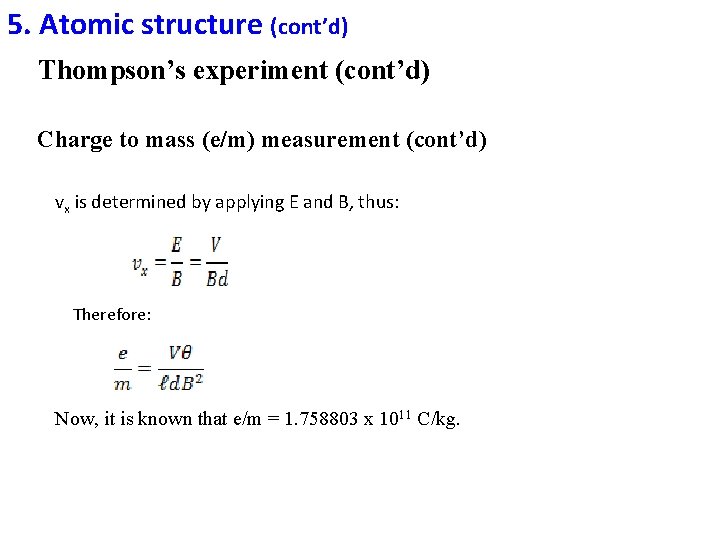
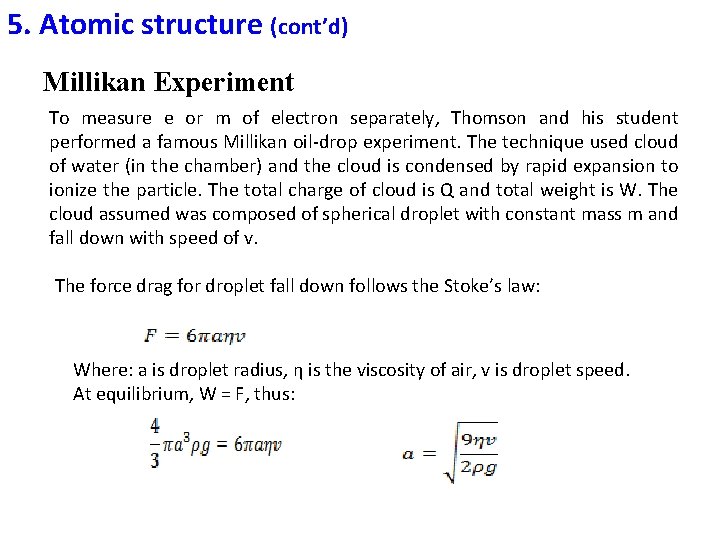
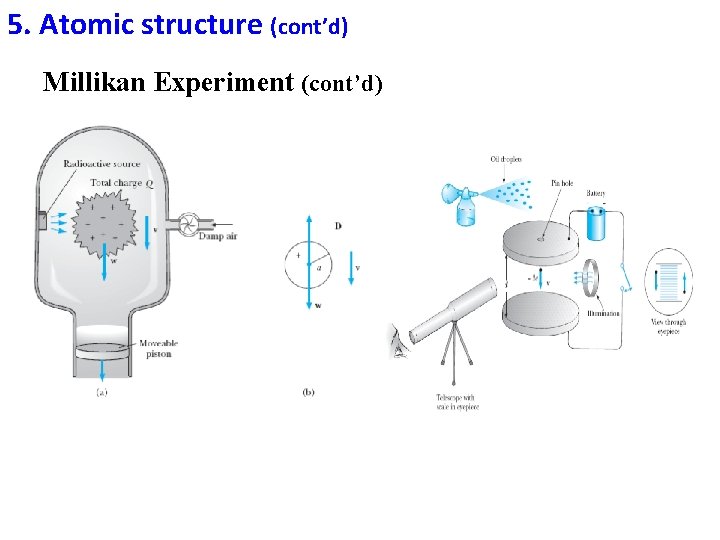
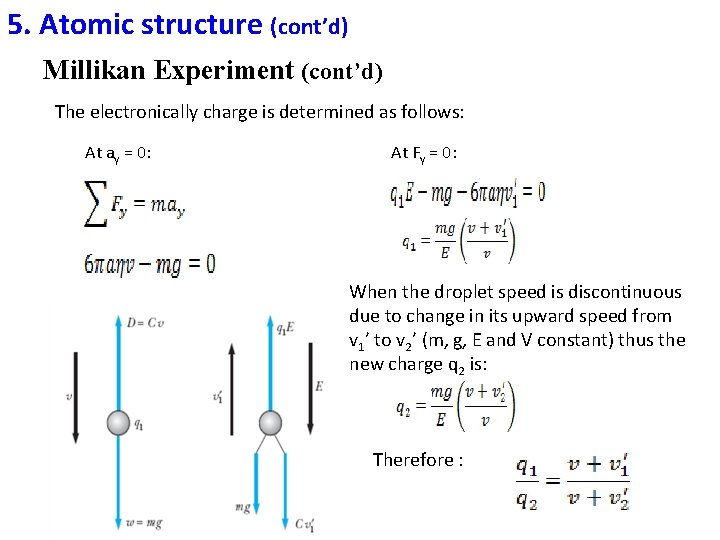
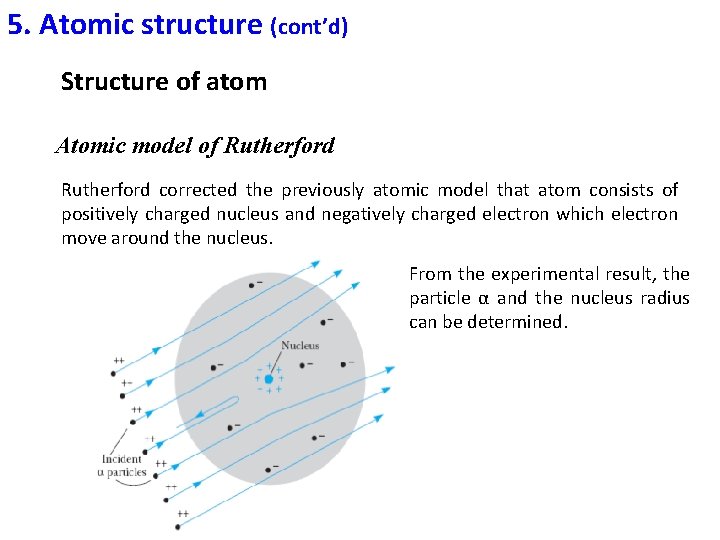
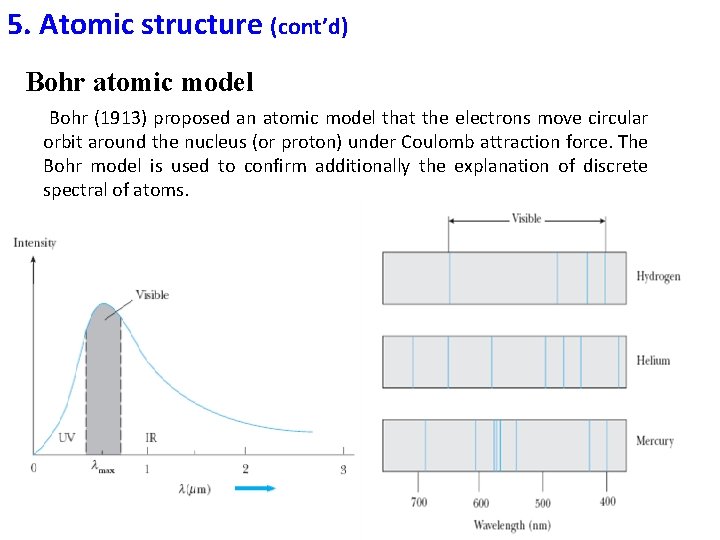
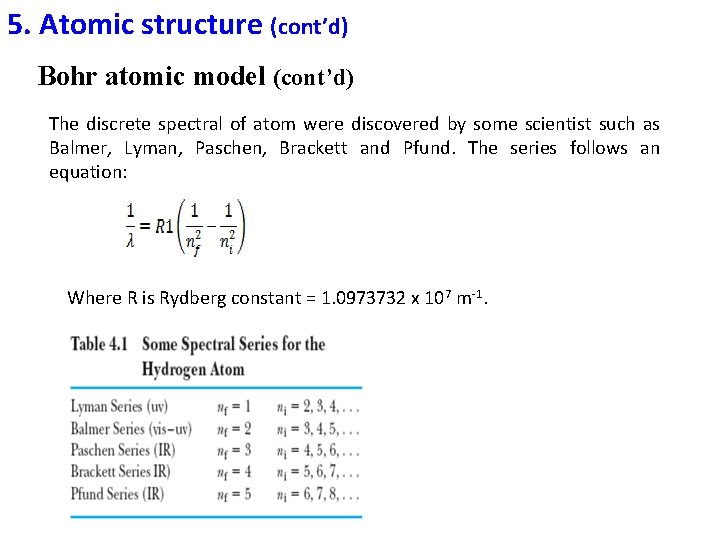
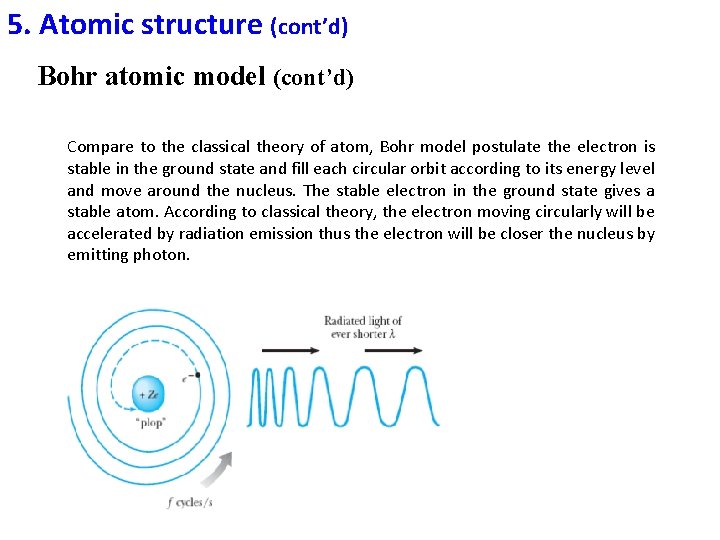
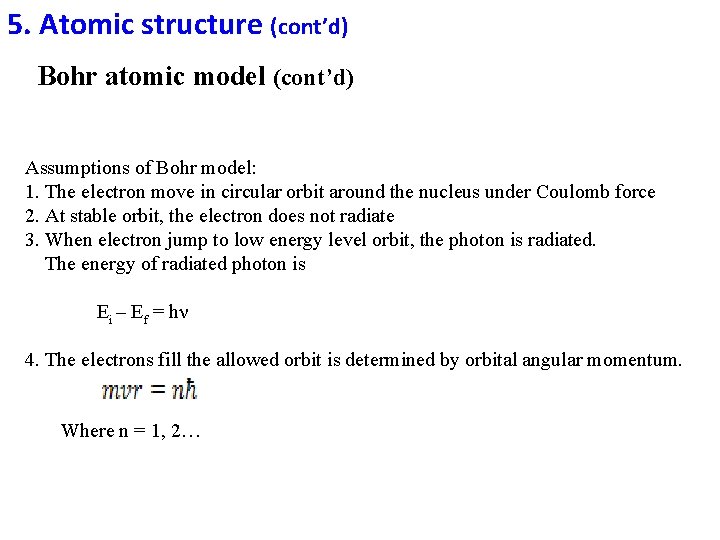
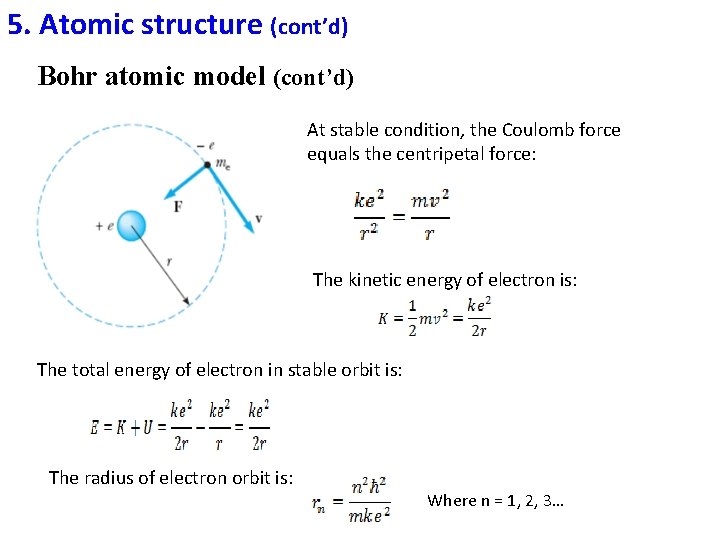
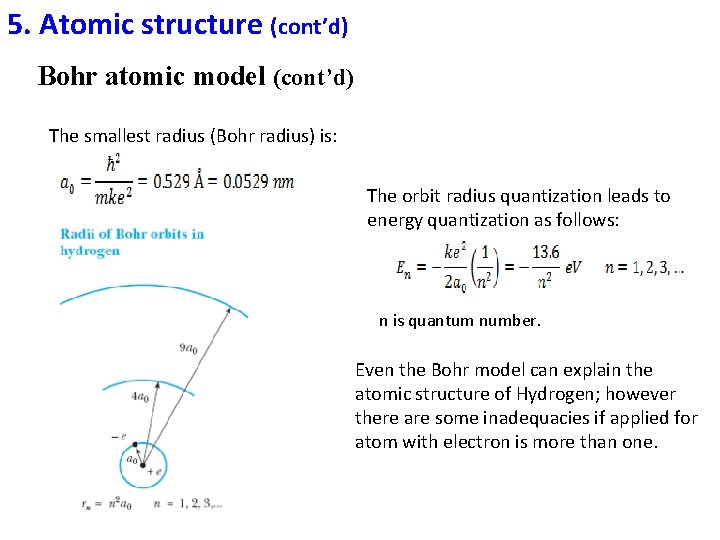
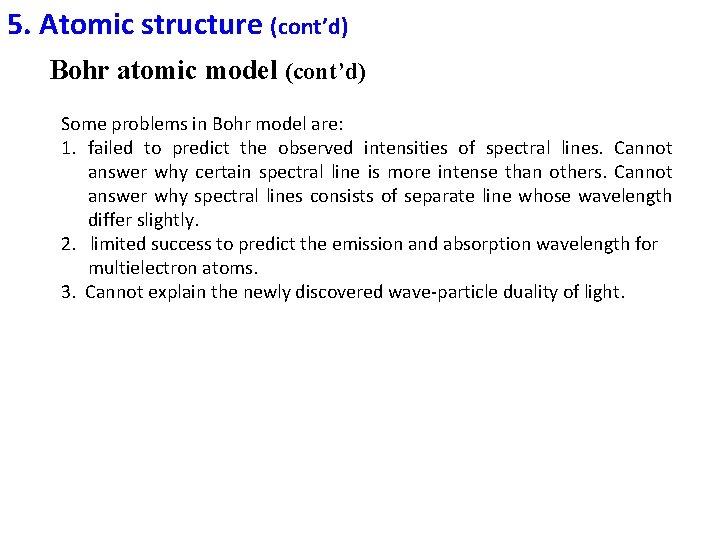
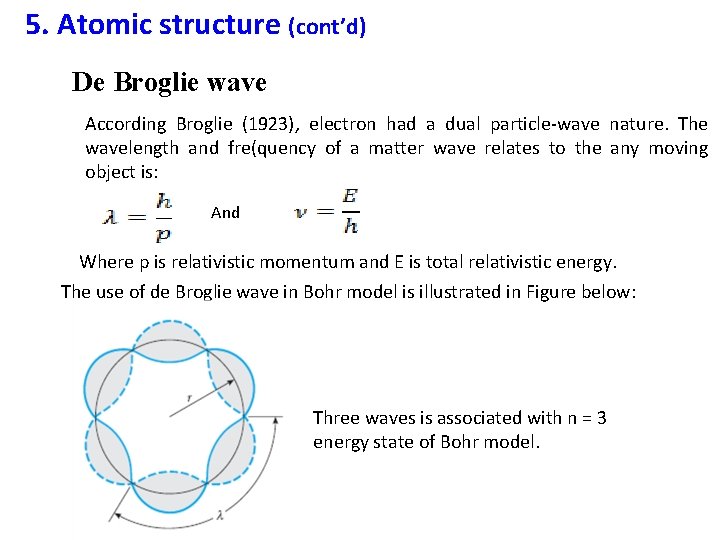
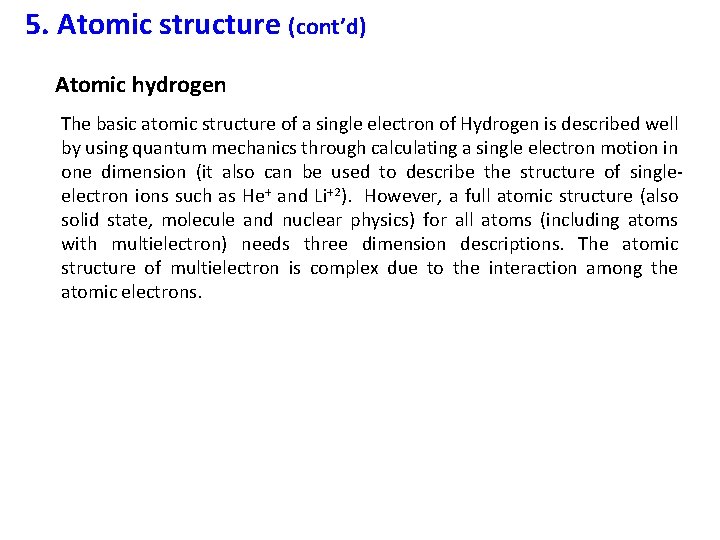
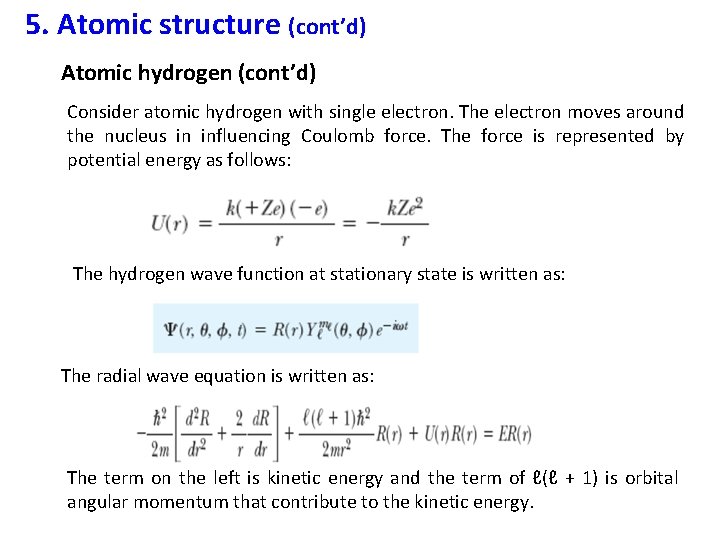
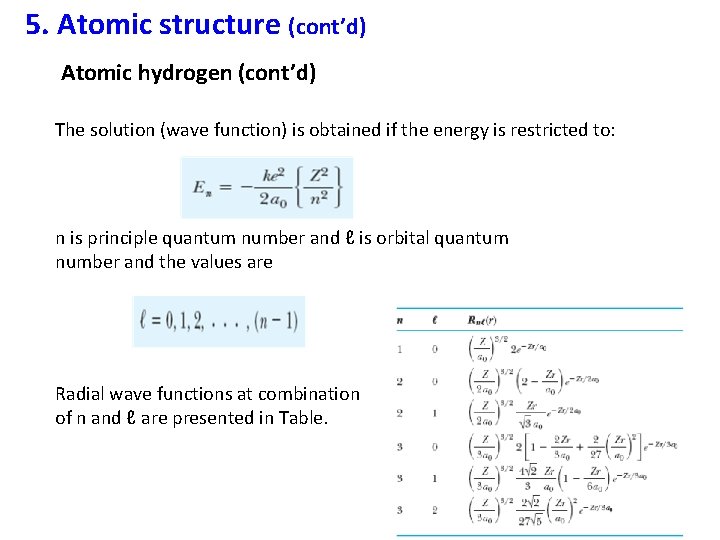
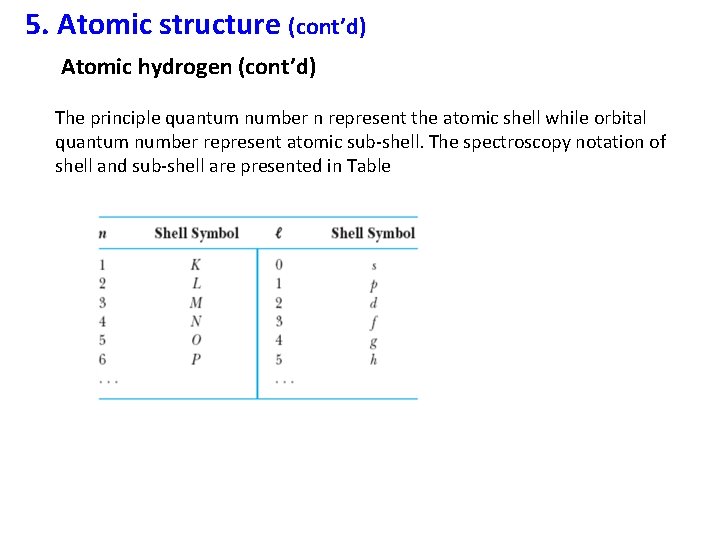
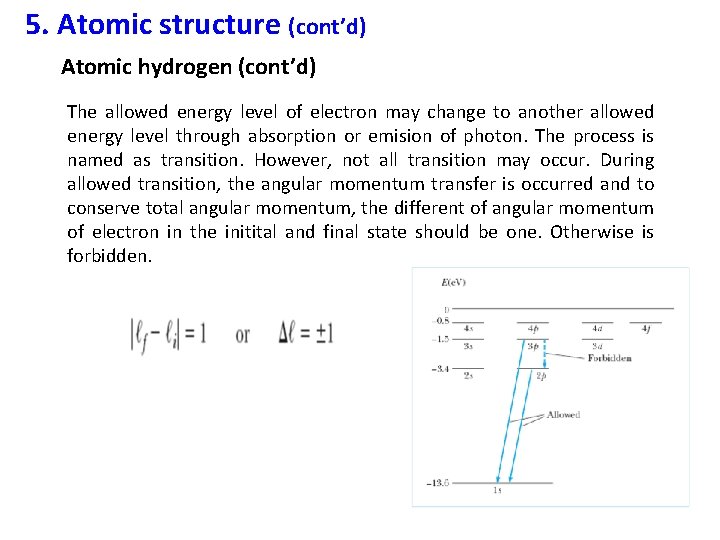
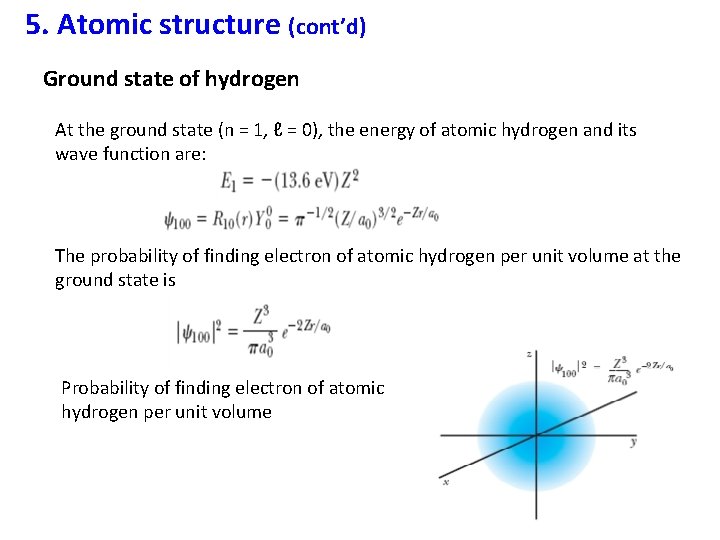
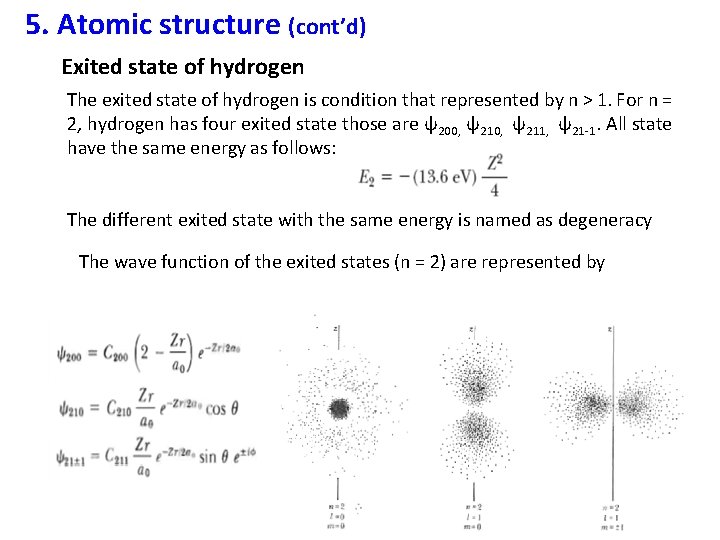
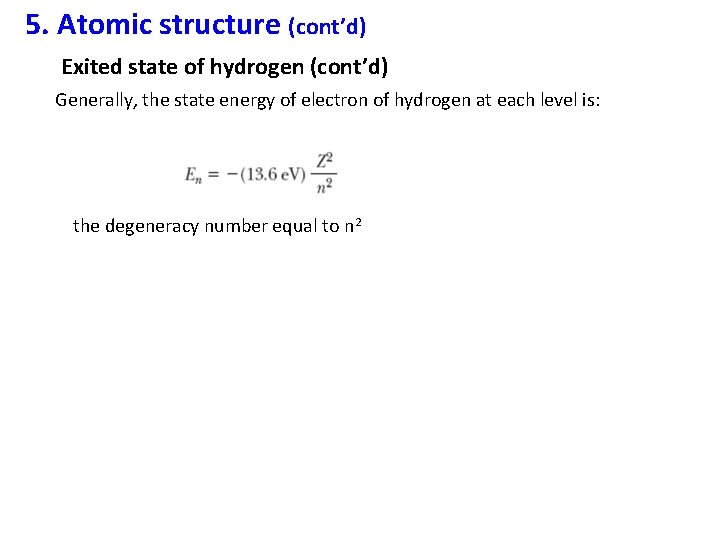
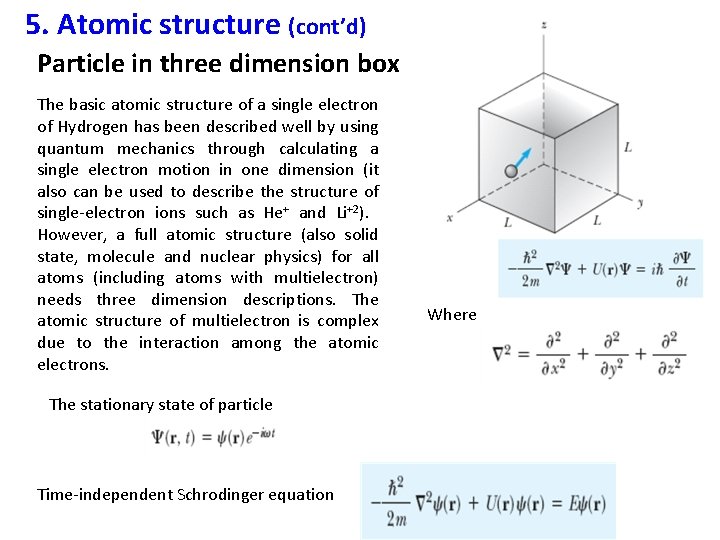
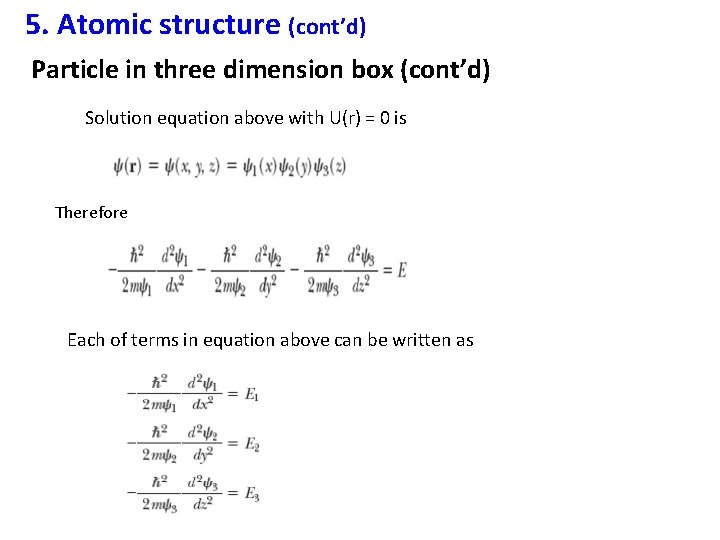
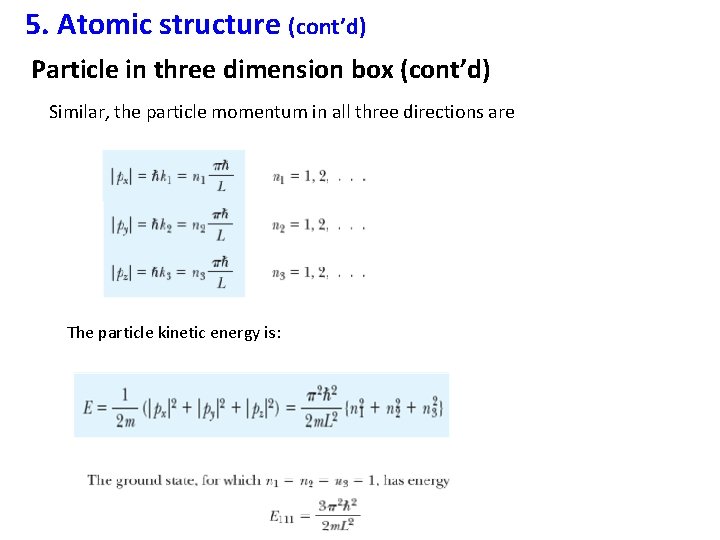
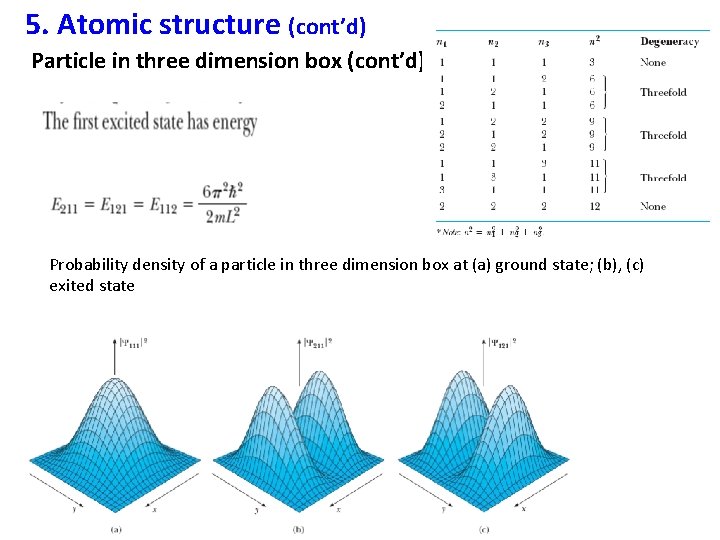
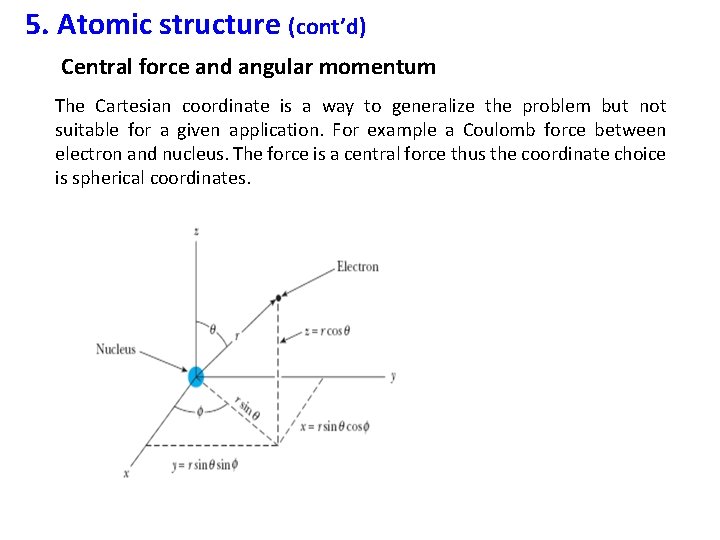
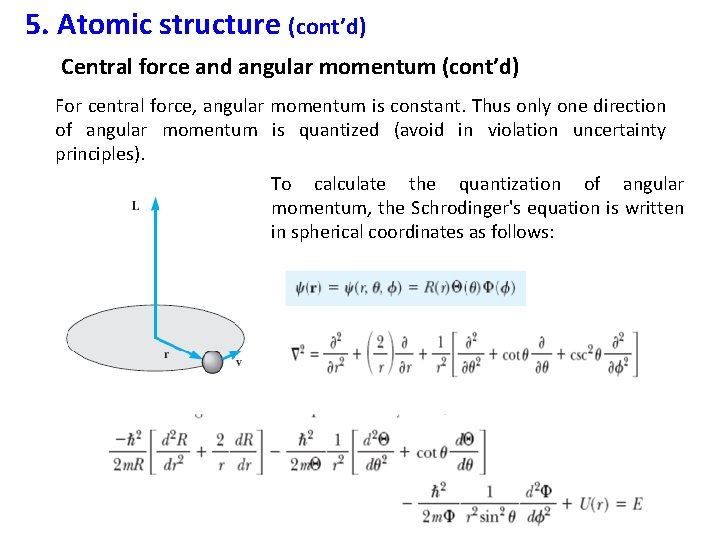
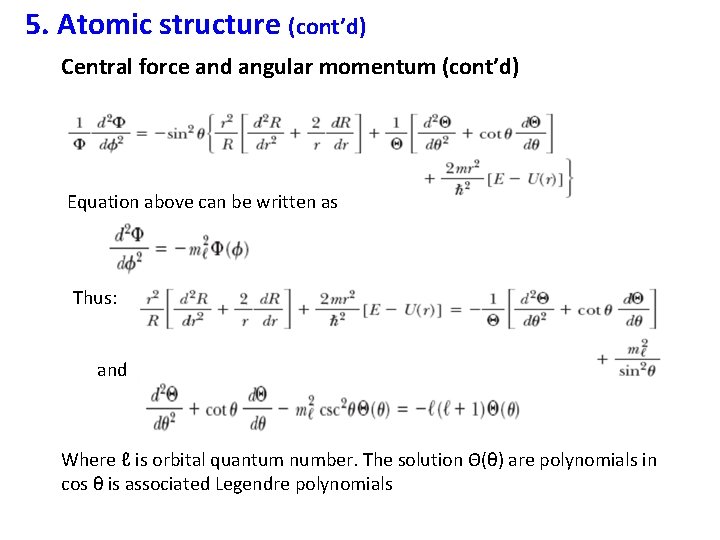
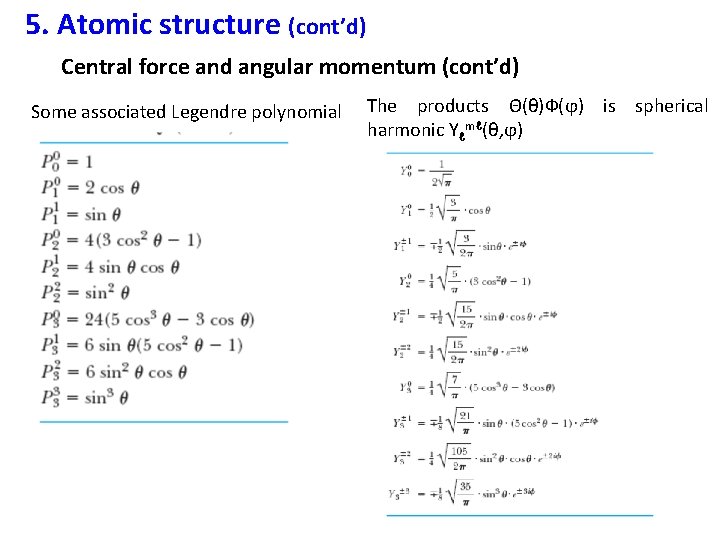
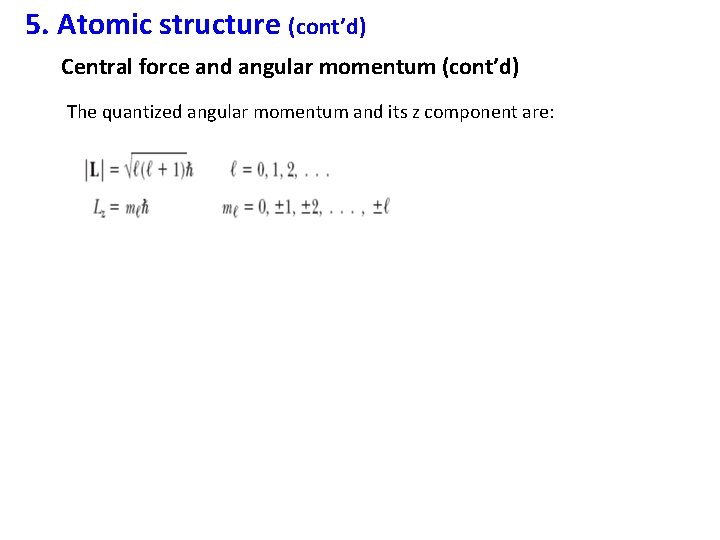
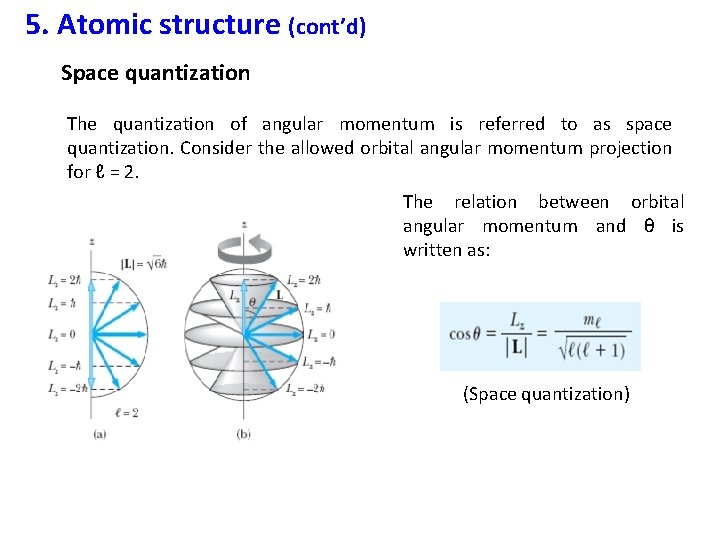
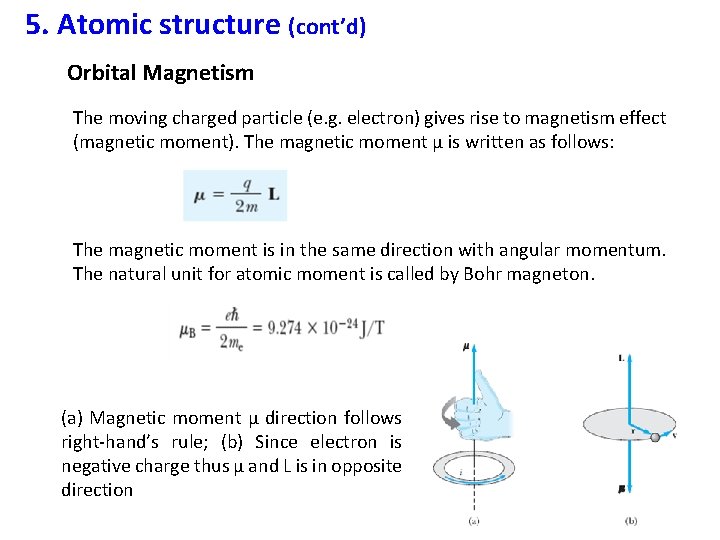
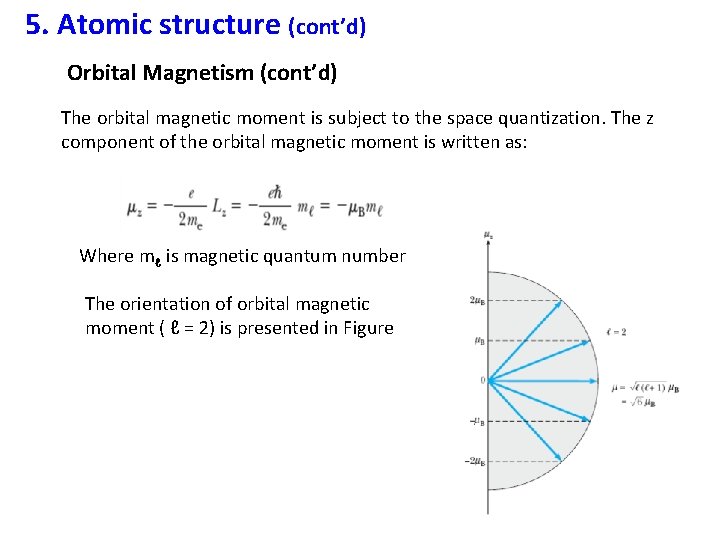
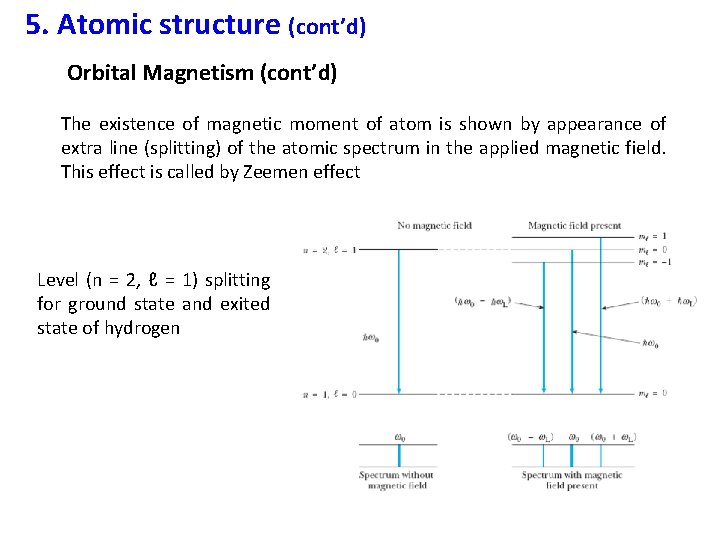
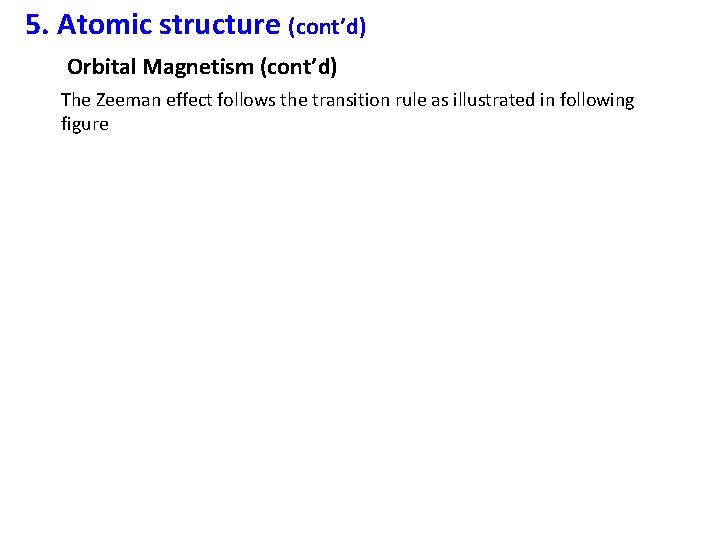
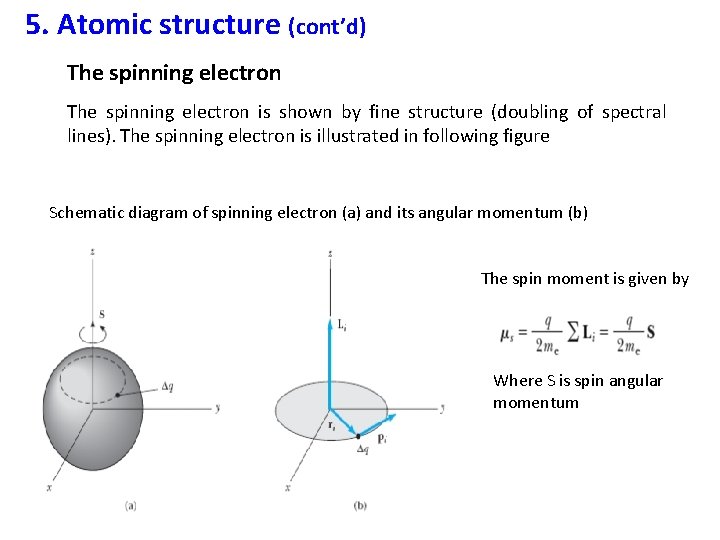
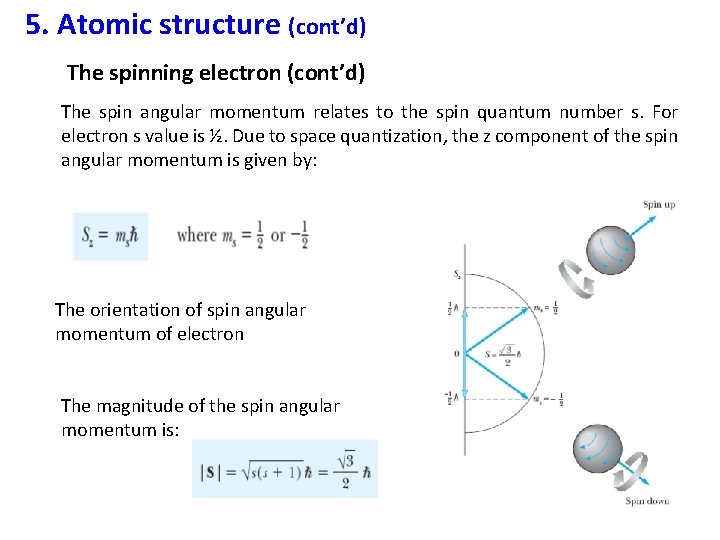
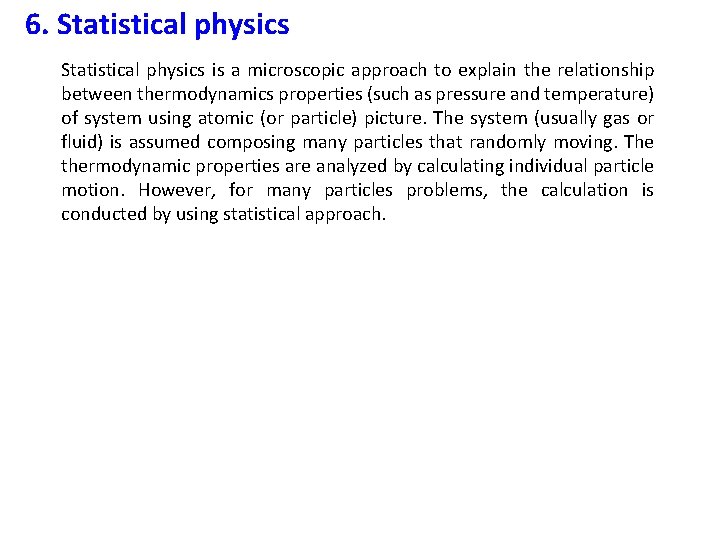
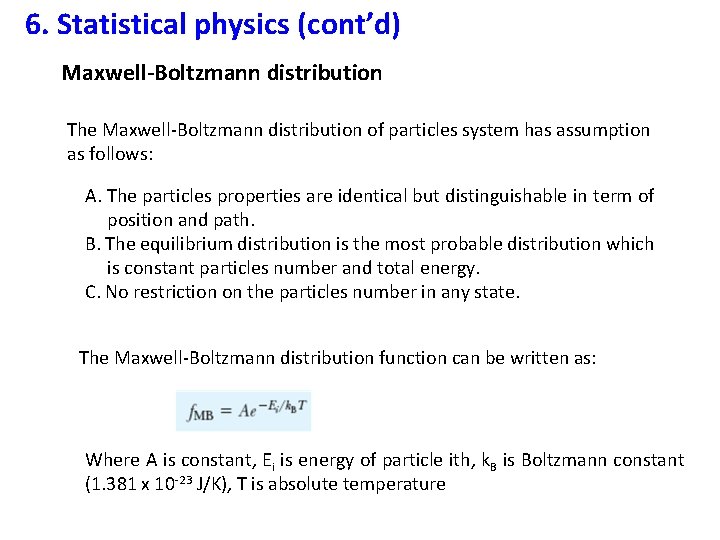
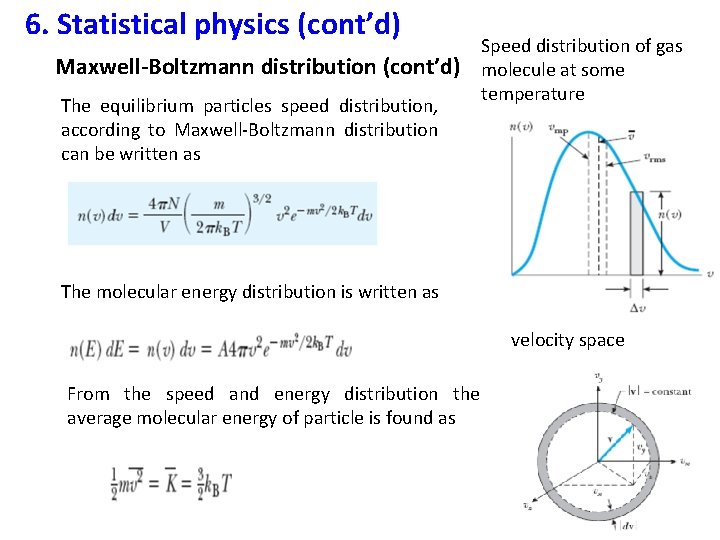
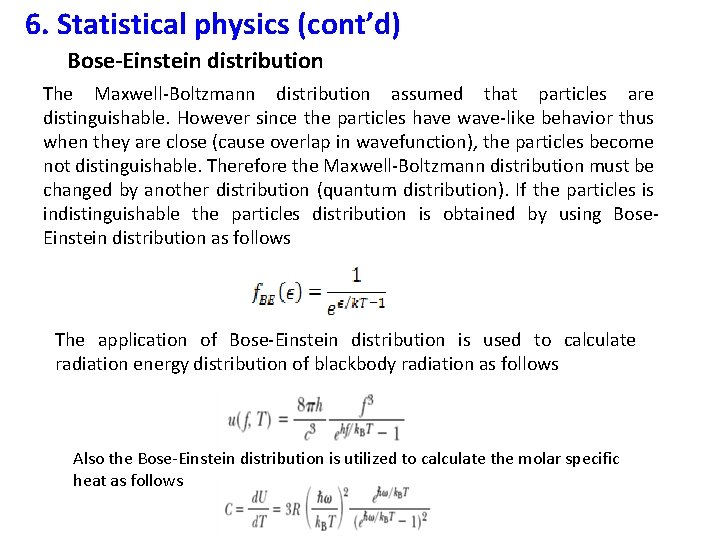
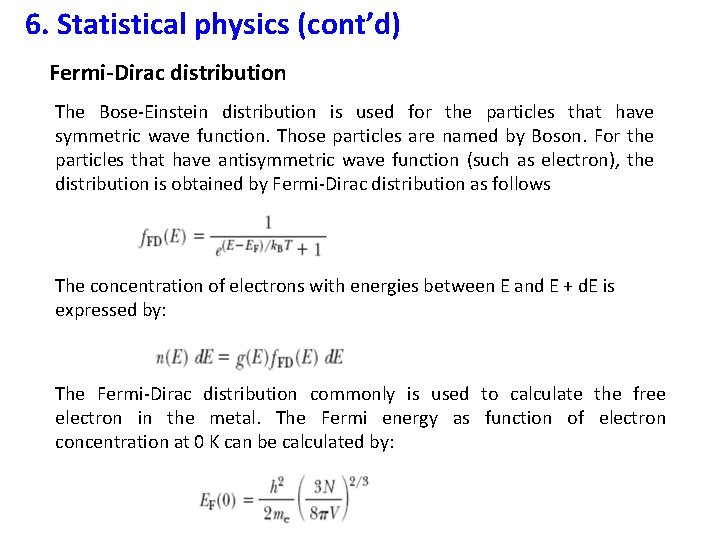
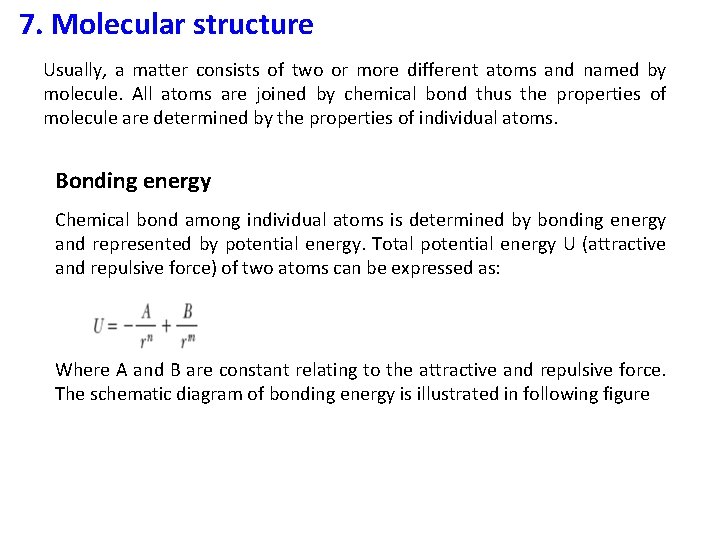
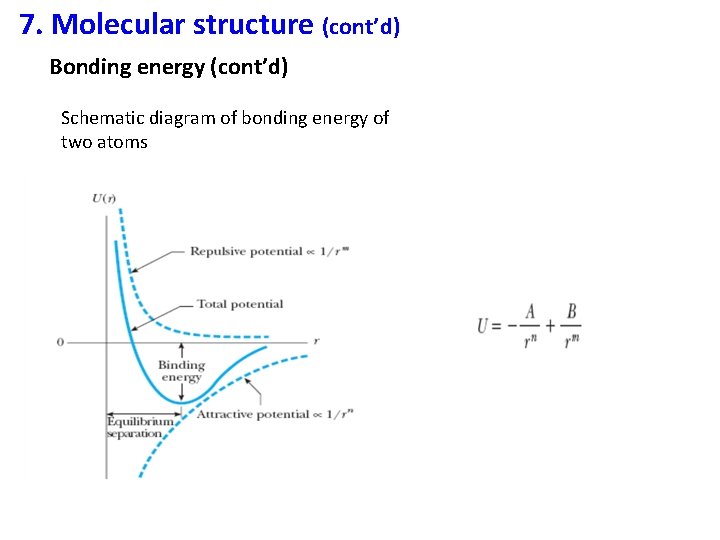
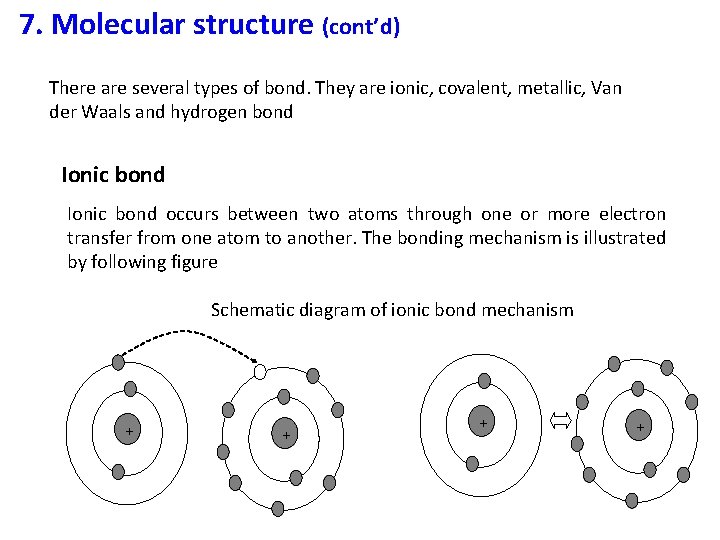
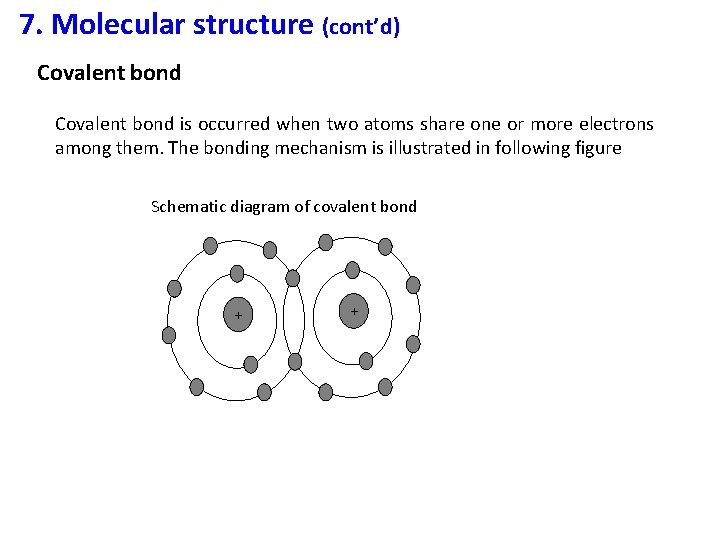
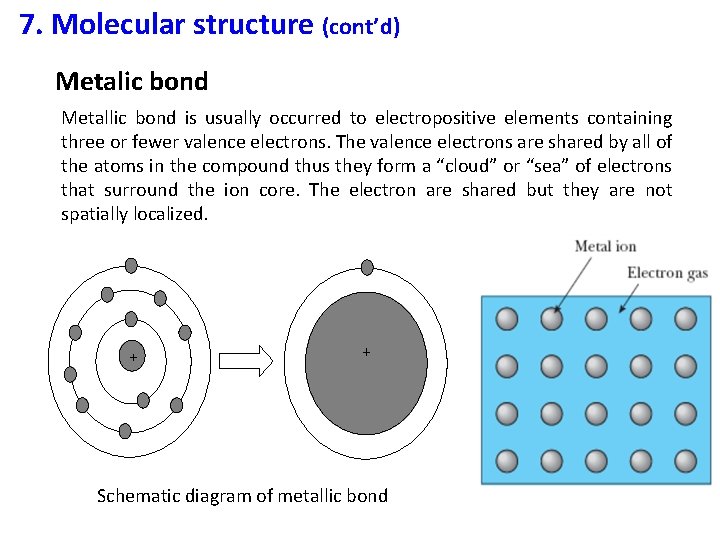
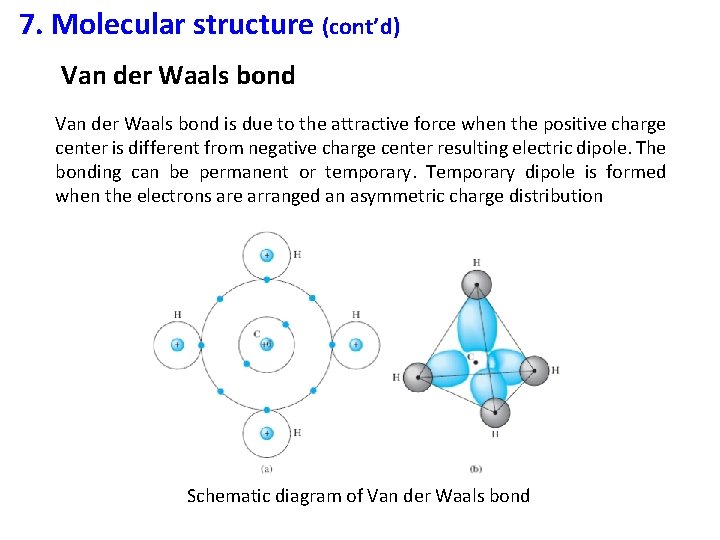
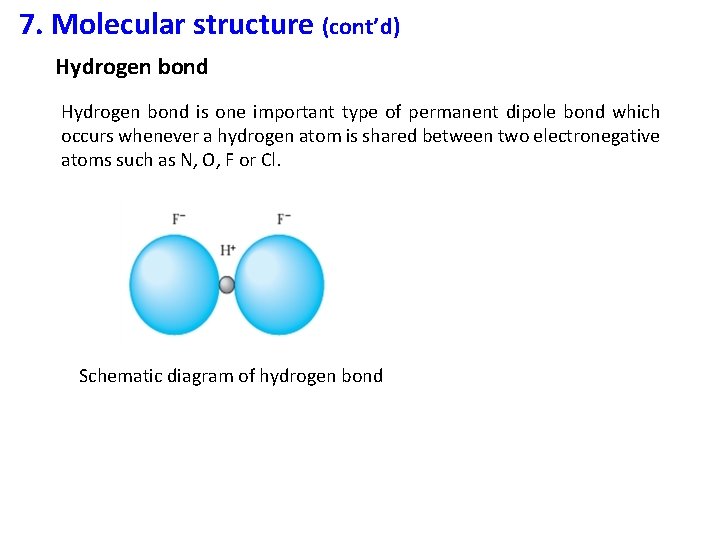
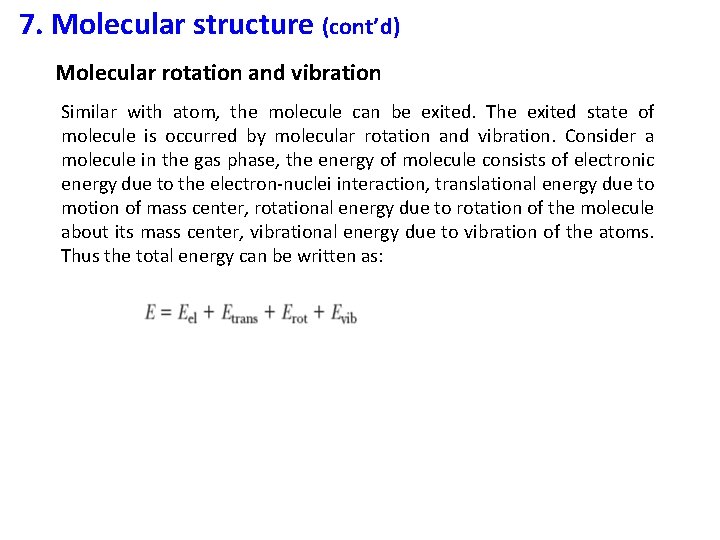
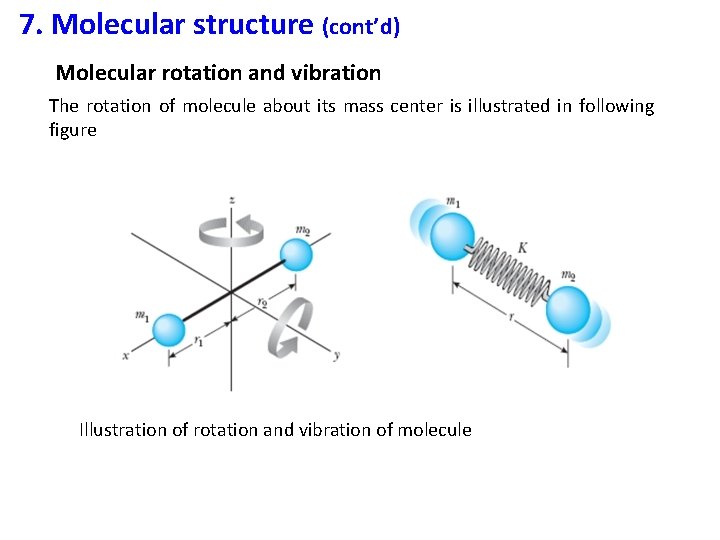
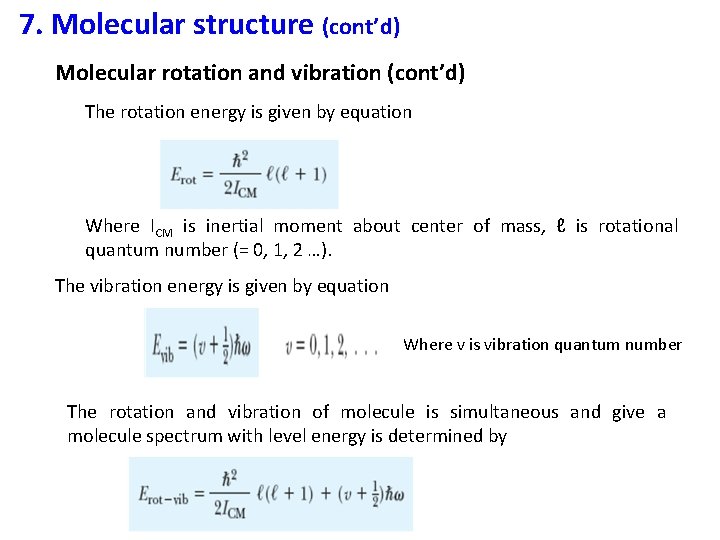
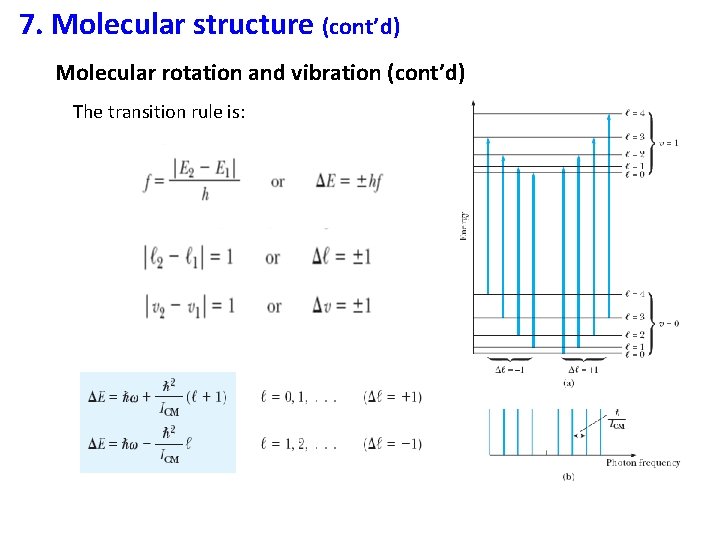
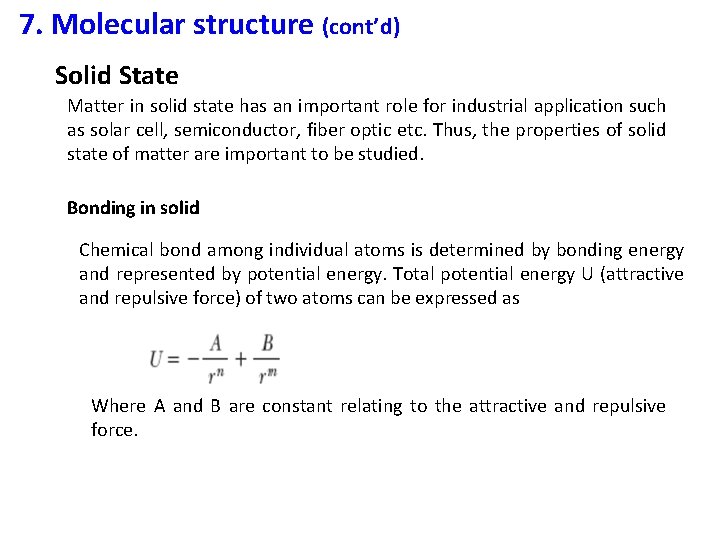
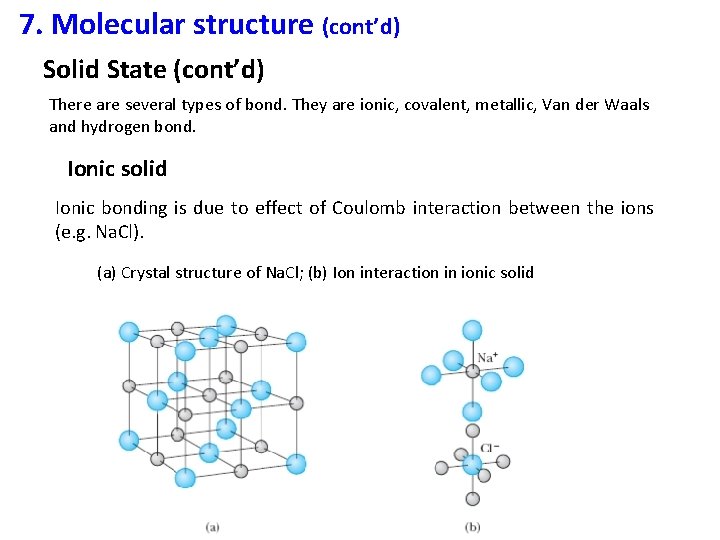
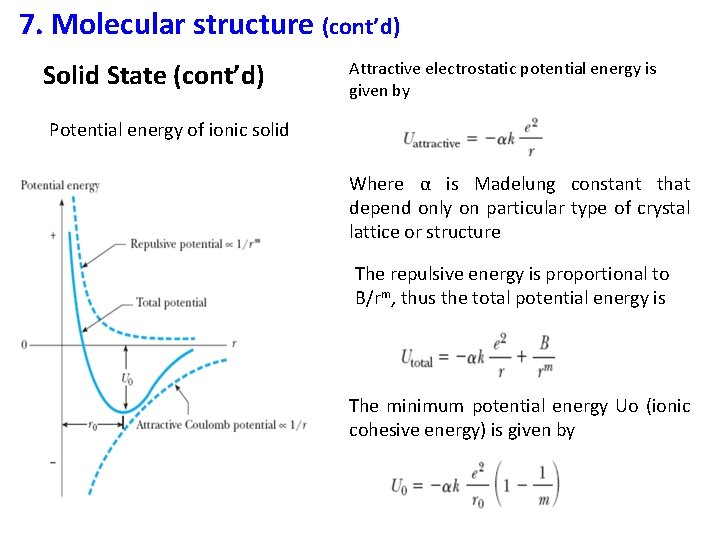
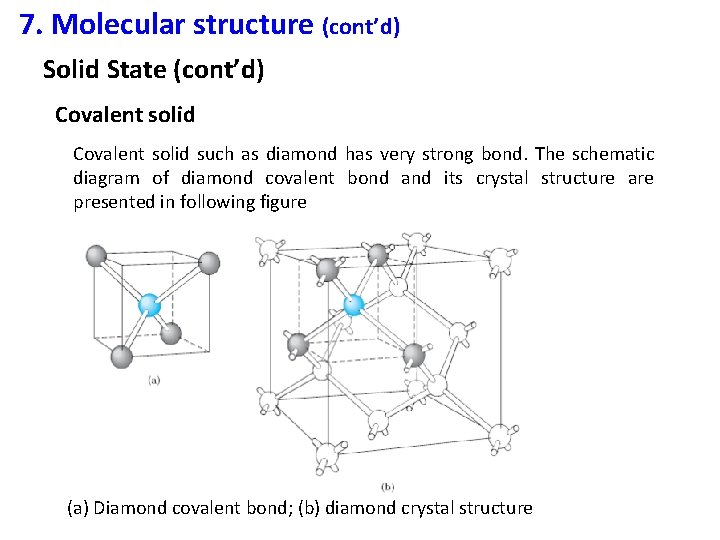
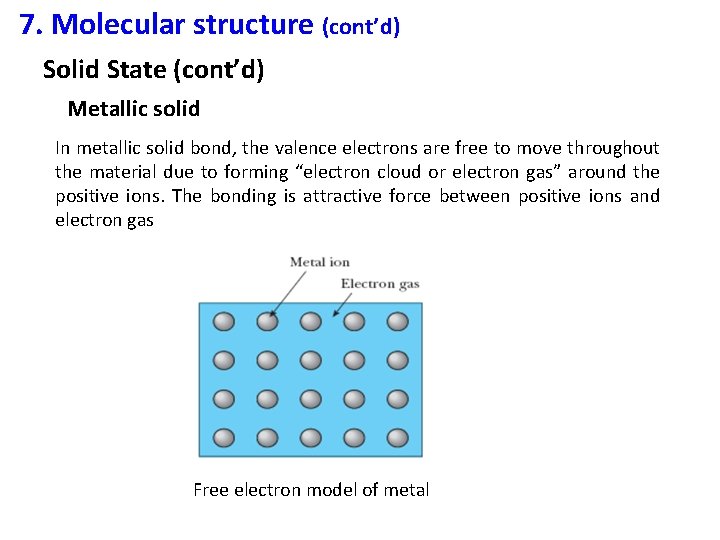
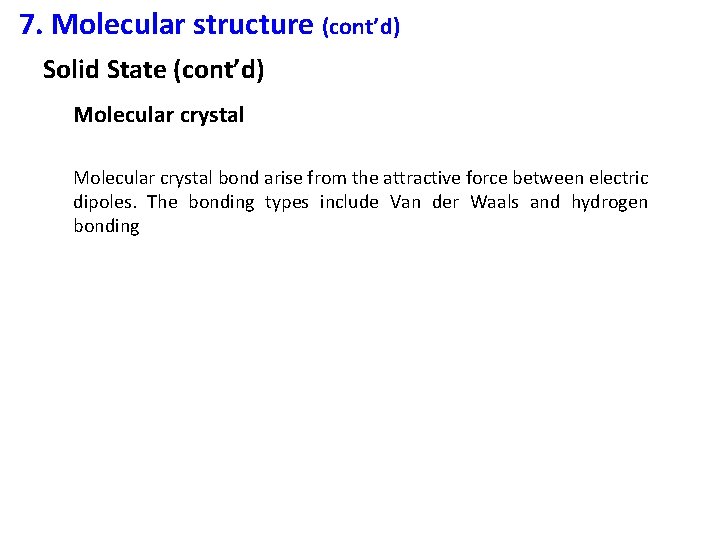
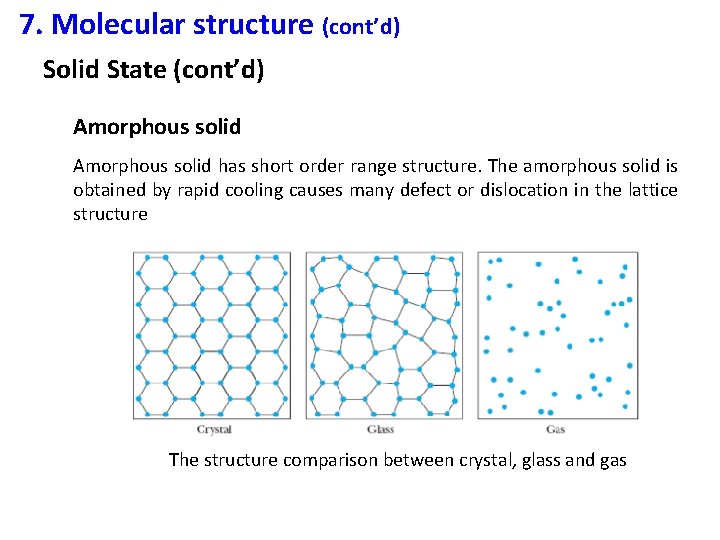
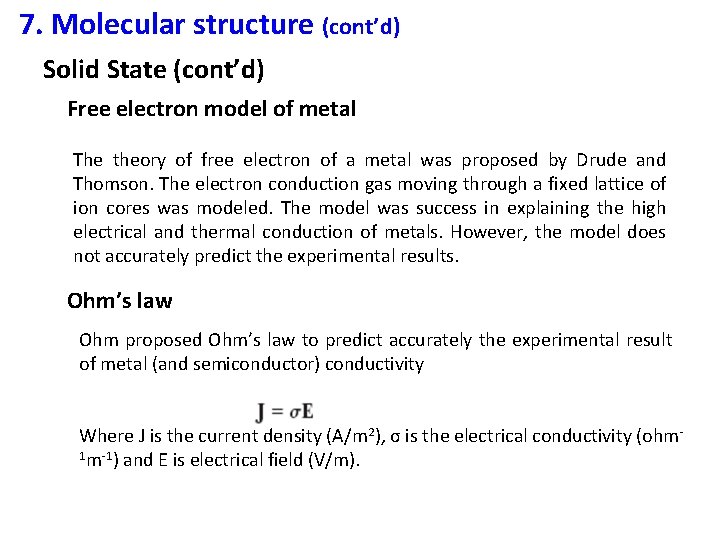
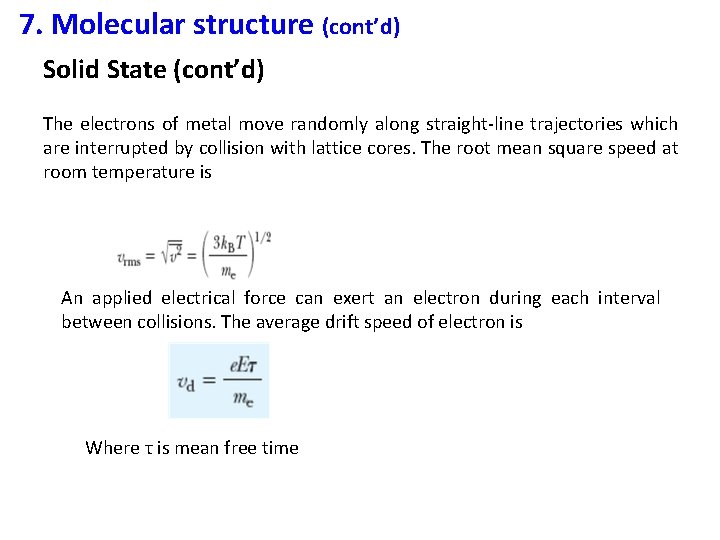
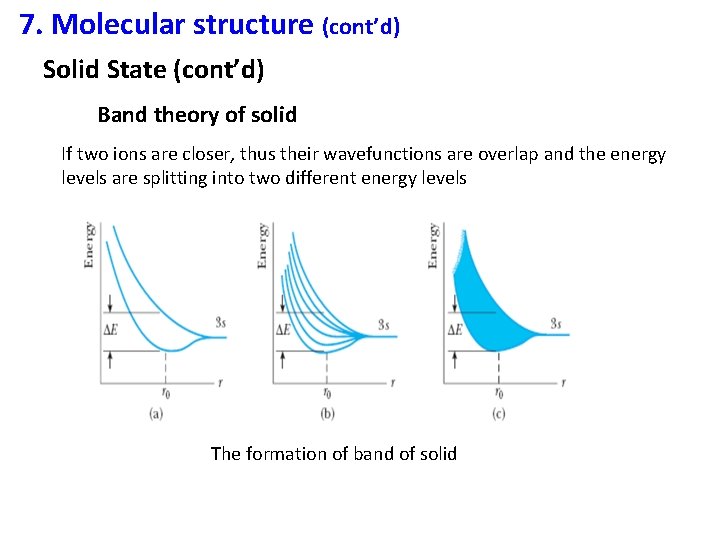
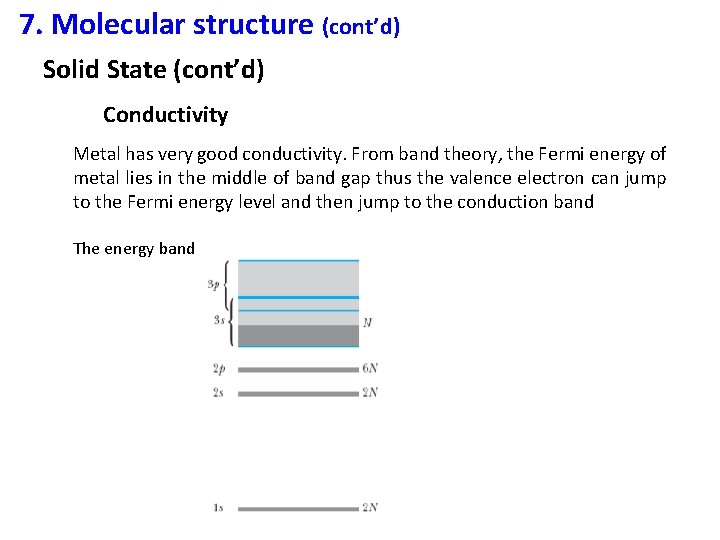
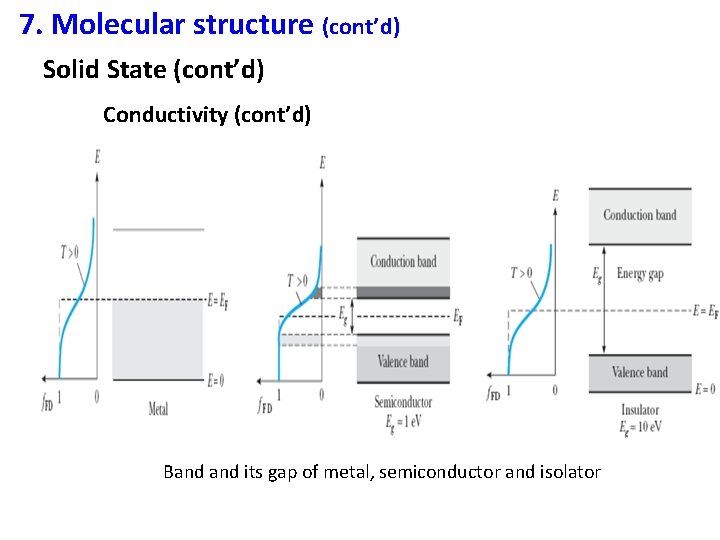
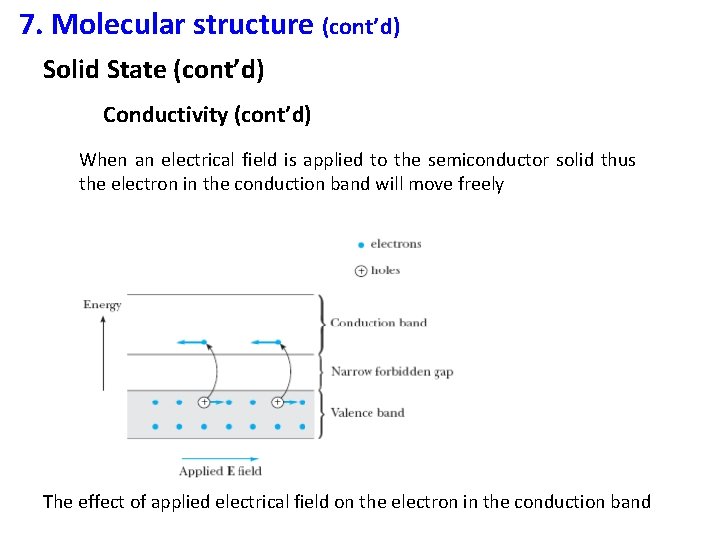
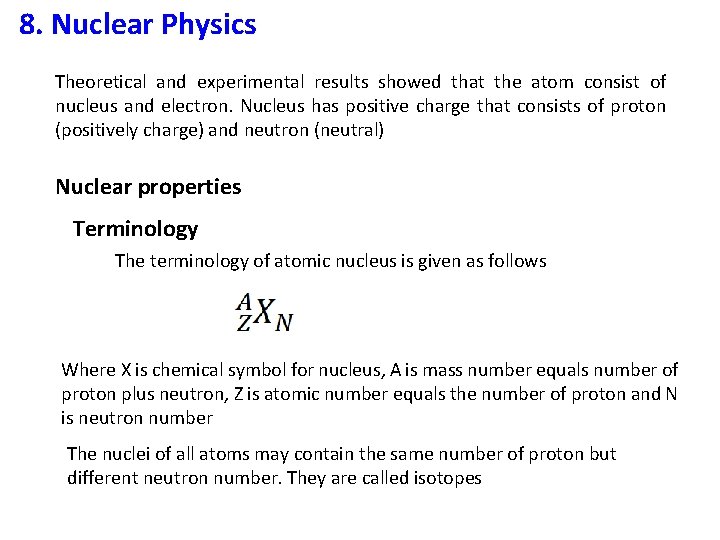
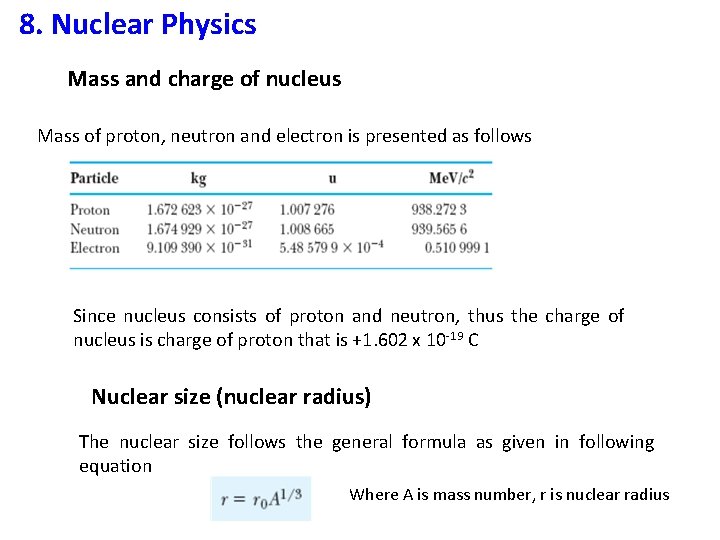
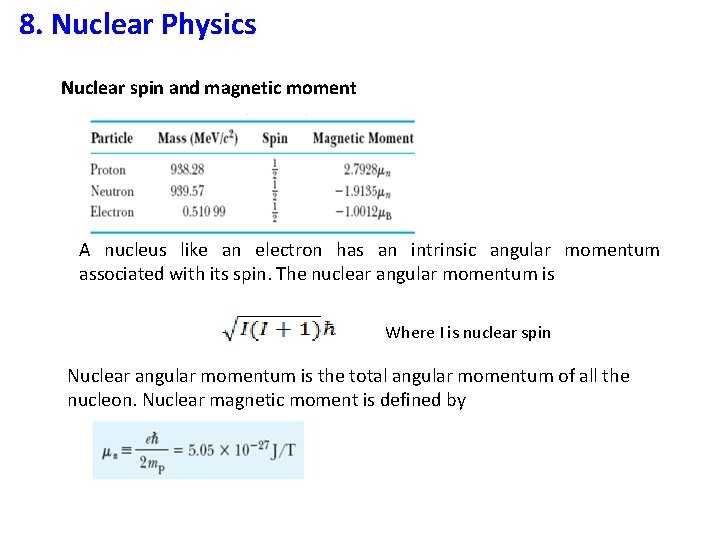
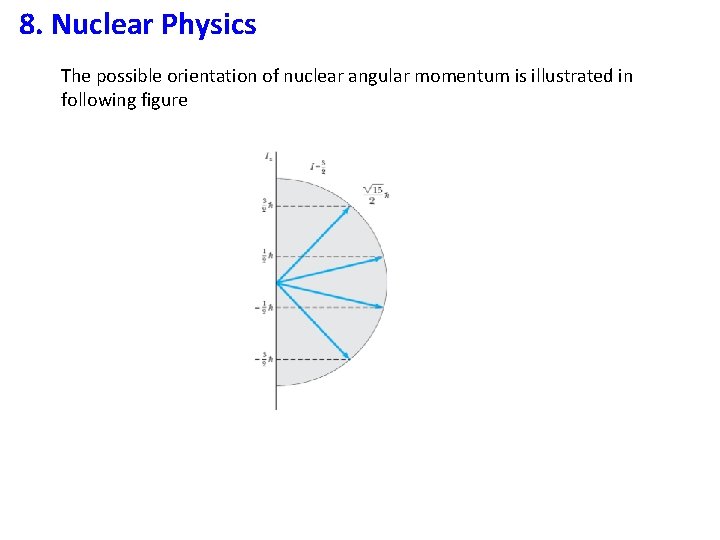
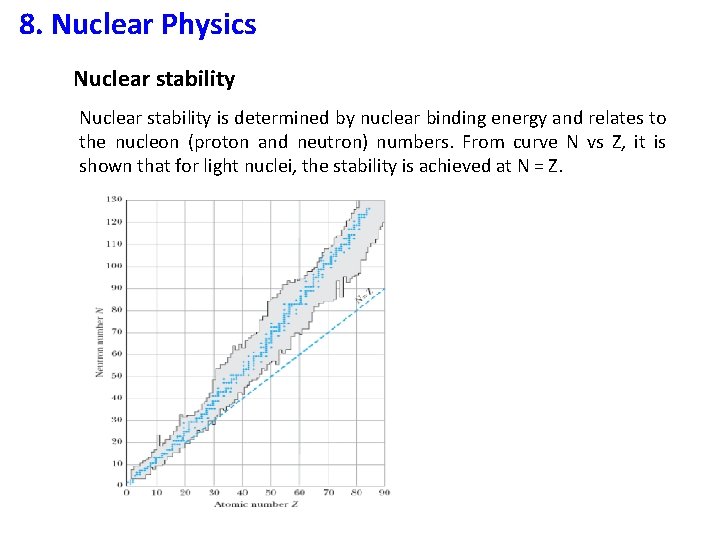
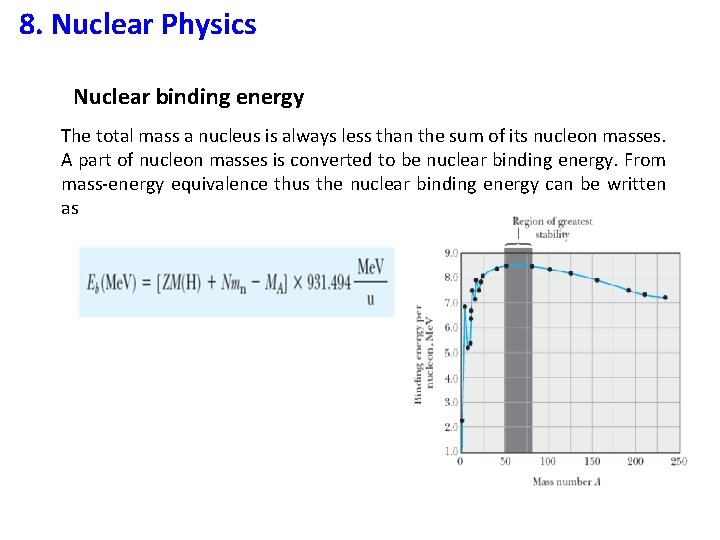
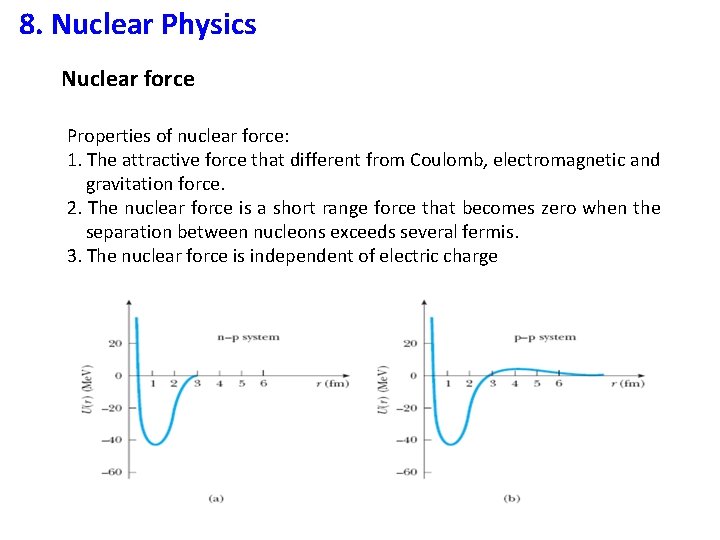
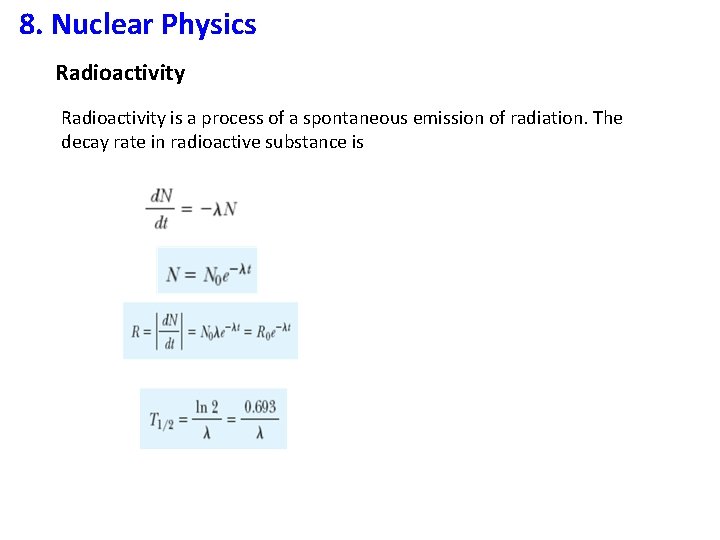
- Slides: 155
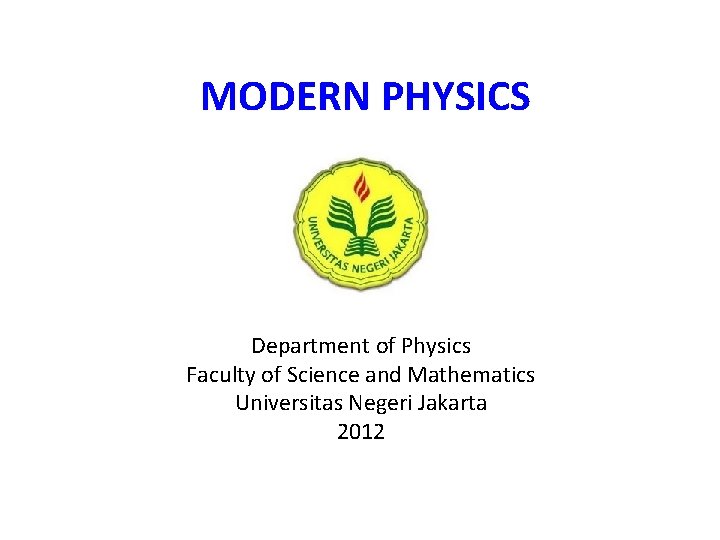
MODERN PHYSICS Department of Physics Faculty of Science and Mathematics Universitas Negeri Jakarta 2012
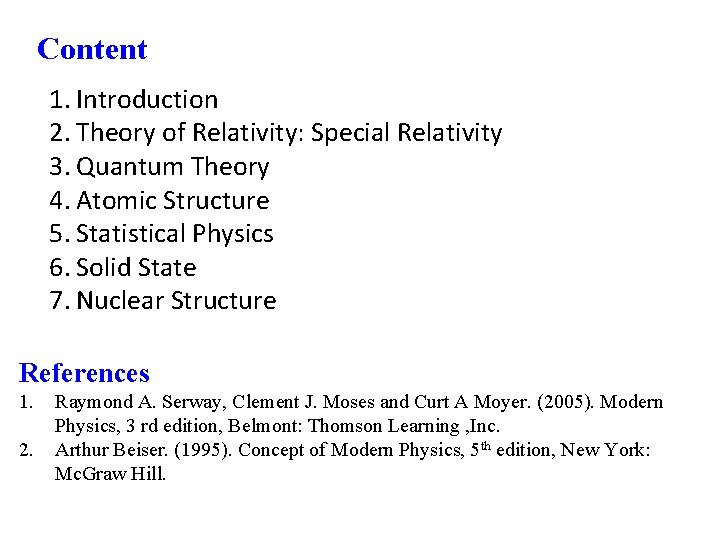
Content 1. Introduction 2. Theory of Relativity: Special Relativity 3. Quantum Theory 4. Atomic Structure 5. Statistical Physics 6. Solid State 7. Nuclear Structure References 1. 2. Raymond A. Serway, Clement J. Moses and Curt A Moyer. (2005). Modern Physics, 3 rd edition, Belmont: Thomson Learning , Inc. Arthur Beiser. (1995). Concept of Modern Physics, 5 th edition, New York: Mc. Graw Hill.
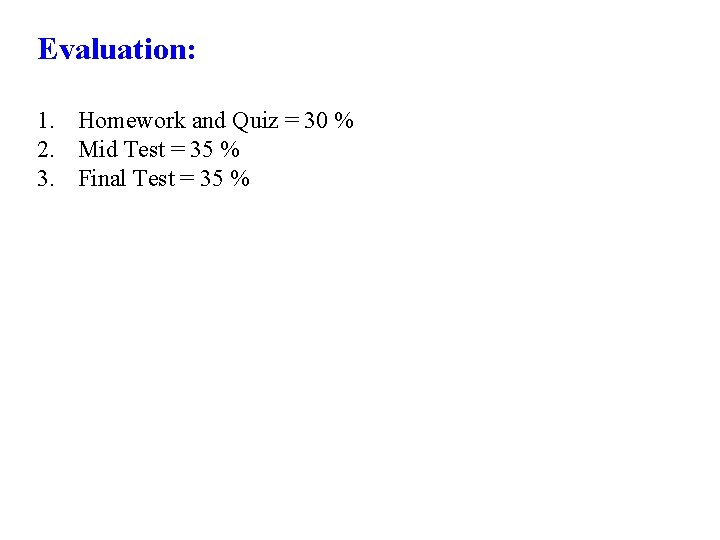
Evaluation: 1. Homework and Quiz = 30 % 2. Mid Test = 35 % 3. Final Test = 35 %
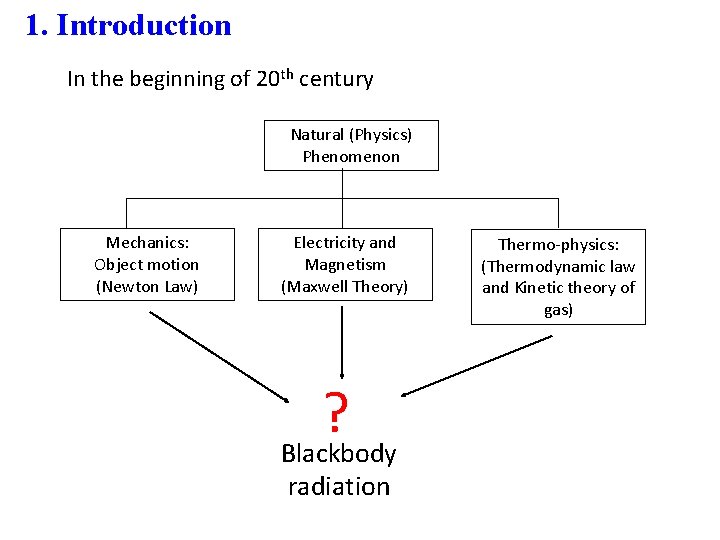
1. Introduction In the beginning of 20 th century Natural (Physics) Phenomenon Mechanics: Object motion (Newton Law) Electricity and Magnetism (Maxwell Theory) ? Blackbody radiation Thermo-physics: (Thermodynamic law and Kinetic theory of gas)
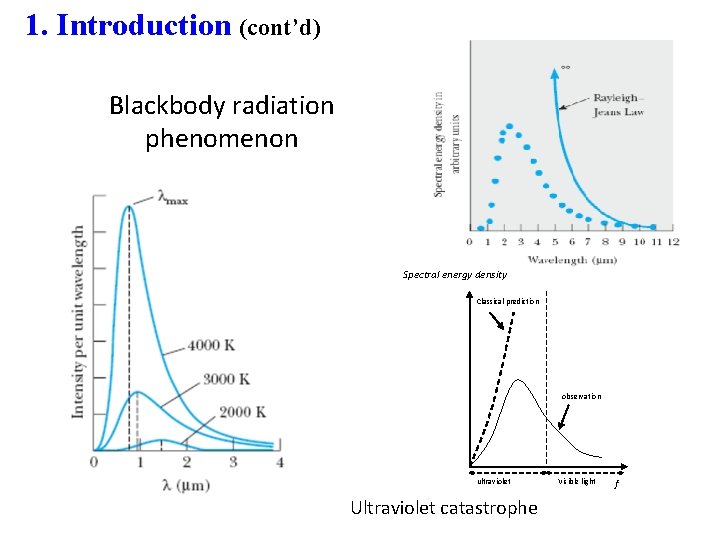
1. Introduction (cont’d) Blackbody radiation phenomenon Spectral energy density Classical prediction observation ultraviolet Ultraviolet catastrophe Visible light f
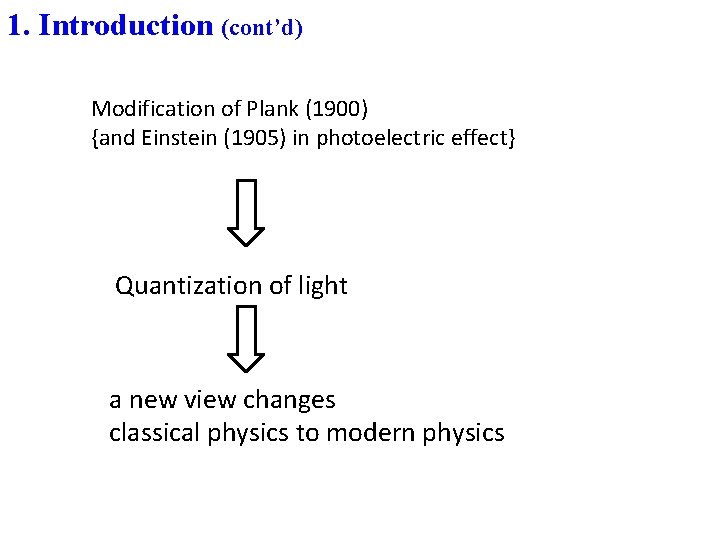
1. Introduction (cont’d) Modification of Plank (1900) {and Einstein (1905) in photoelectric effect} Quantization of light a new view changes classical physics to modern physics
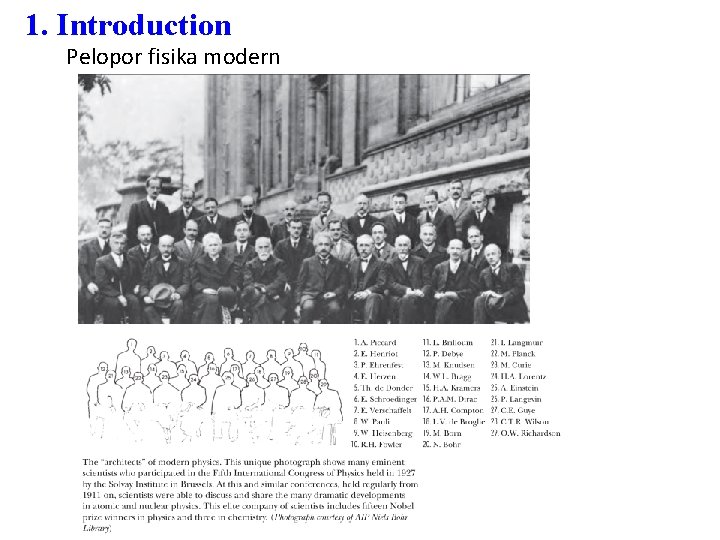
1. Introduction Pelopor fisika modern
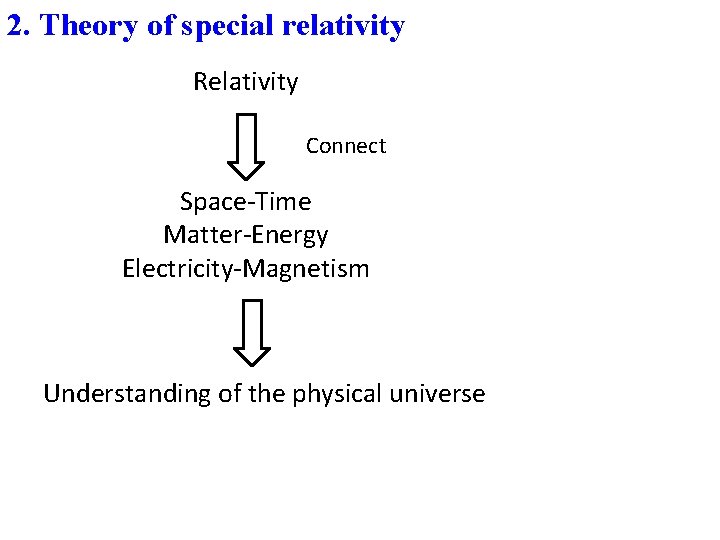
2. Theory of special relativity Relativity Connect Space-Time Matter-Energy Electricity-Magnetism Understanding of the physical universe
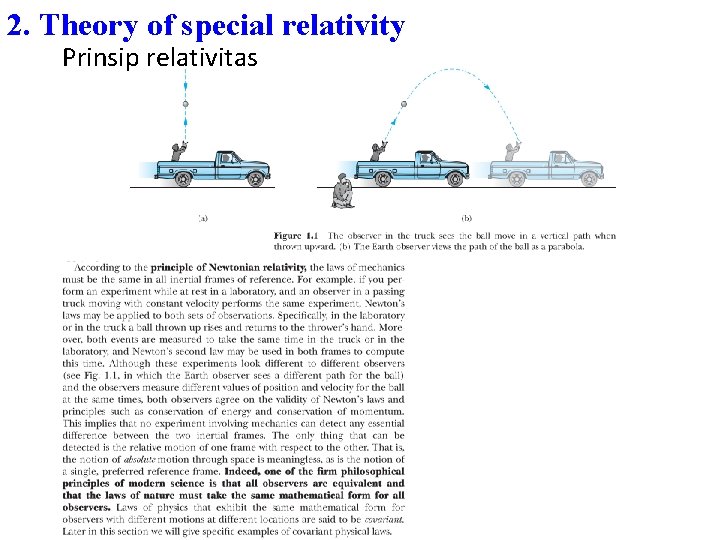
2. Theory of special relativity Prinsip relativitas
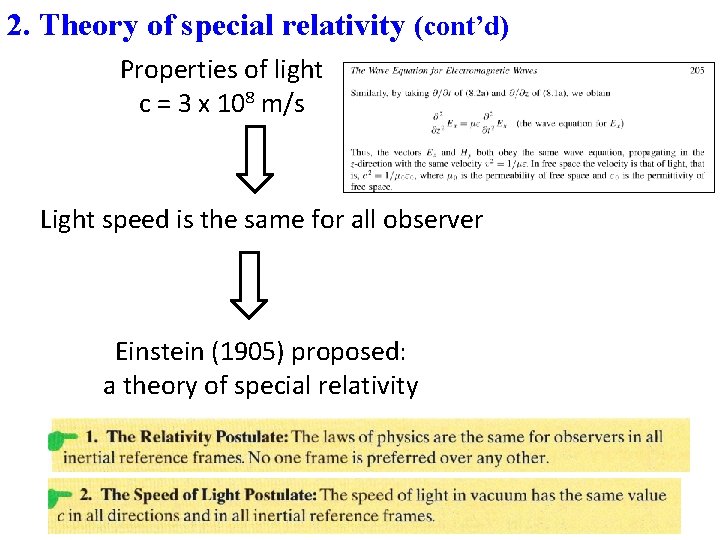
2. Theory of special relativity (cont’d) Properties of light c = 3 x 108 m/s Light speed is the same for all observer Einstein (1905) proposed: a theory of special relativity
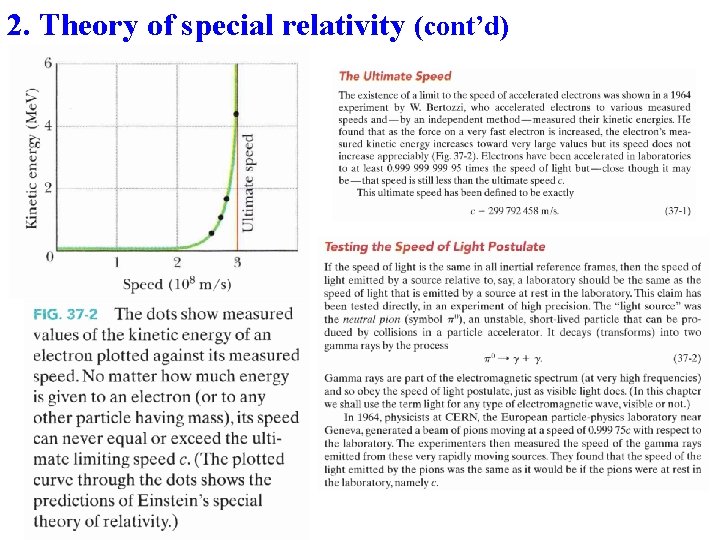
2. Theory of special relativity (cont’d)
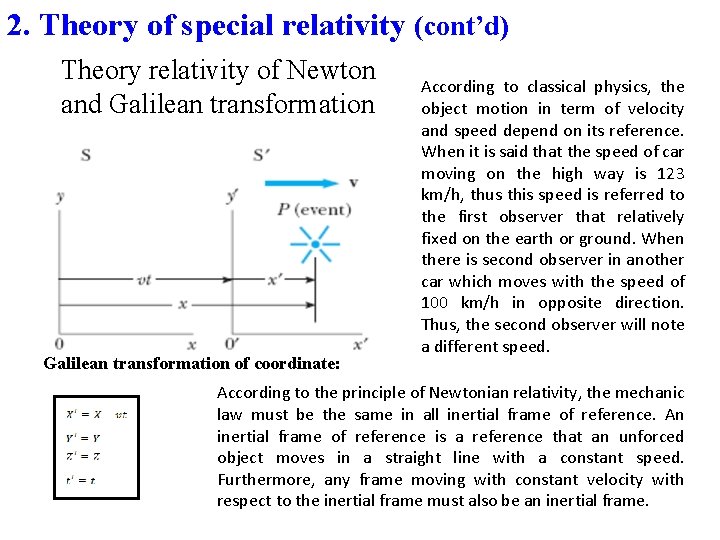
2. Theory of special relativity (cont’d) Theory relativity of Newton and Galilean transformation of coordinate: According to classical physics, the object motion in term of velocity and speed depend on its reference. When it is said that the speed of car moving on the high way is 123 km/h, thus this speed is referred to the first observer that relatively fixed on the earth or ground. When there is second observer in another car which moves with the speed of 100 km/h in opposite direction. Thus, the second observer will note a different speed. According to the principle of Newtonian relativity, the mechanic law must be the same in all inertial frame of reference. An inertial frame of reference is a reference that an unforced object moves in a straight line with a constant speed. Furthermore, any frame moving with constant velocity with respect to the inertial frame must also be an inertial frame.
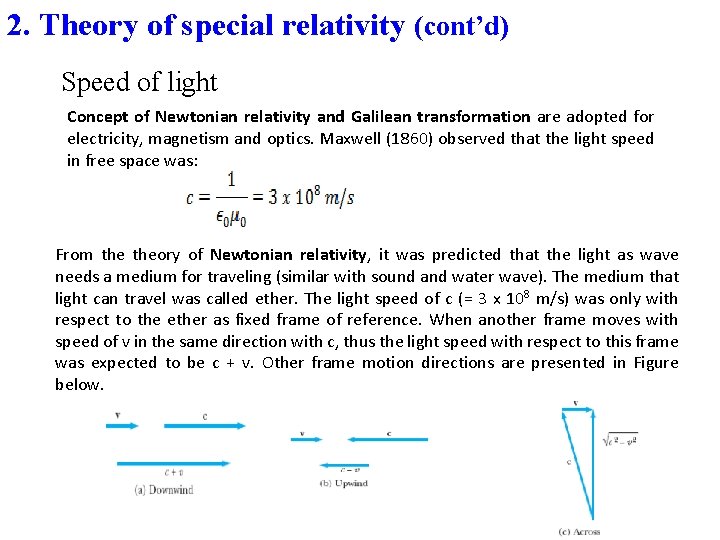
2. Theory of special relativity (cont’d) Speed of light Concept of Newtonian relativity and Galilean transformation are adopted for electricity, magnetism and optics. Maxwell (1860) observed that the light speed in free space was: From theory of Newtonian relativity, it was predicted that the light as wave needs a medium for traveling (similar with sound and water wave). The medium that light can travel was called ether. The light speed of c (= 3 x 108 m/s) was only with respect to the ether as fixed frame of reference. When another frame moves with speed of v in the same direction with c, thus the light speed with respect to this frame was expected to be c + v. Other frame motion directions are presented in Figure below.
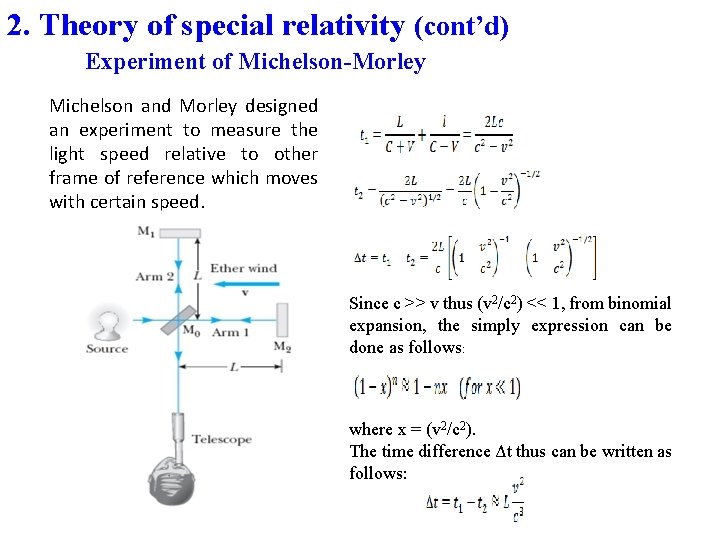
2. Theory of special relativity (cont’d) Experiment of Michelson-Morley Michelson and Morley designed an experiment to measure the light speed relative to other frame of reference which moves with certain speed. Since c >> v thus (v 2/c 2) << 1, from binomial expansion, the simply expression can be done as follows: where x = (v 2/c 2). The time difference Δt thus can be written as follows:
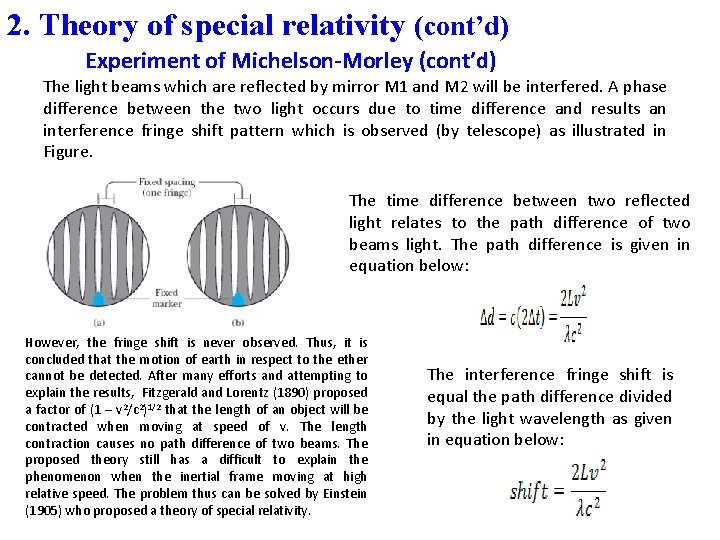
2. Theory of special relativity (cont’d) Experiment of Michelson-Morley (cont’d) The light beams which are reflected by mirror M 1 and M 2 will be interfered. A phase difference between the two light occurs due to time difference and results an interference fringe shift pattern which is observed (by telescope) as illustrated in Figure. The time difference between two reflected light relates to the path difference of two beams light. The path difference is given in equation below: However, the fringe shift is never observed. Thus, it is concluded that the motion of earth in respect to the ether cannot be detected. After many efforts and attempting to explain the results, Fitzgerald and Lorentz (1890) proposed a factor of (1 – v 2/c 2)1/2 that the length of an object will be contracted when moving at speed of v. The length contraction causes no path difference of two beams. The proposed theory still has a difficult to explain the phenomenon when the inertial frame moving at high relative speed. The problem thus can be solved by Einstein (1905) who proposed a theory of special relativity. The interference fringe shift is equal the path difference divided by the light wavelength as given in equation below:
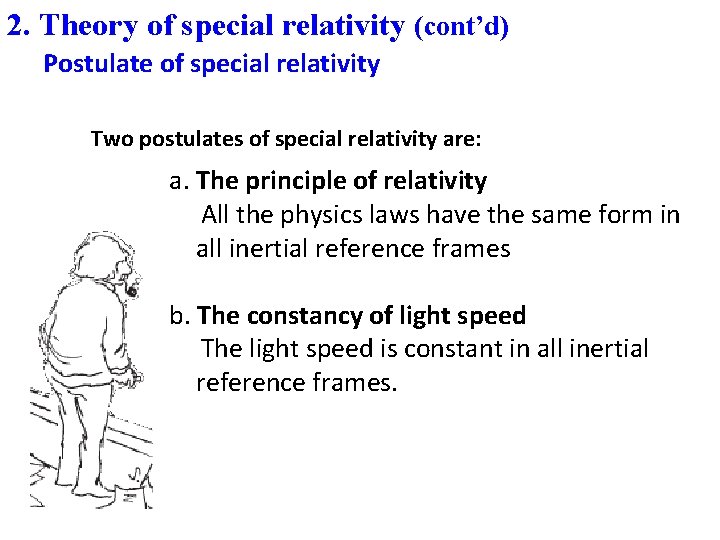
2. Theory of special relativity (cont’d) Postulate of special relativity Two postulates of special relativity are: a. The principle of relativity All the physics laws have the same form in all inertial reference frames b. The constancy of light speed The light speed is constant in all inertial reference frames.
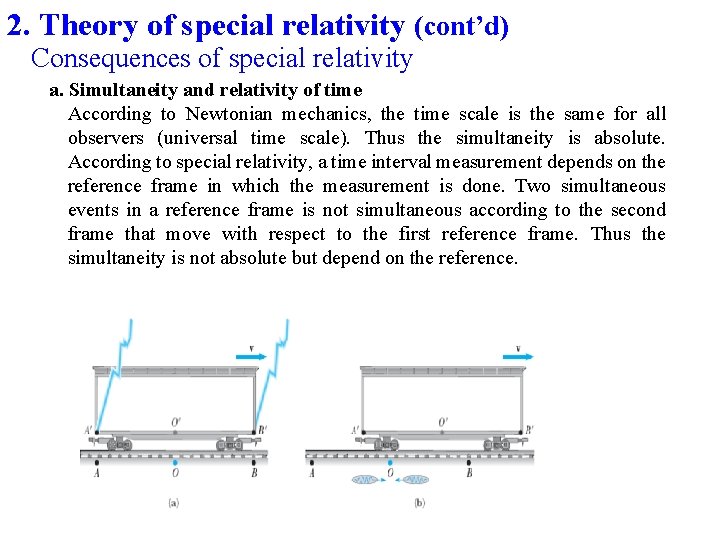
2. Theory of special relativity (cont’d) Consequences of special relativity a. Simultaneity and relativity of time According to Newtonian mechanics, the time scale is the same for all observers (universal time scale). Thus the simultaneity is absolute. According to special relativity, a time interval measurement depends on the reference frame in which the measurement is done. Two simultaneous events in a reference frame is not simultaneous according to the second frame that move with respect to the first reference frame. Thus the simultaneity is not absolute but depend on the reference.
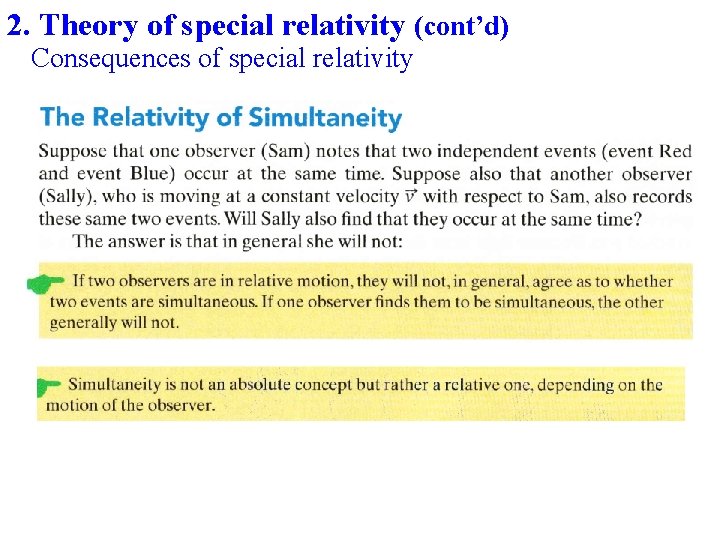
2. Theory of special relativity (cont’d) Consequences of special relativity
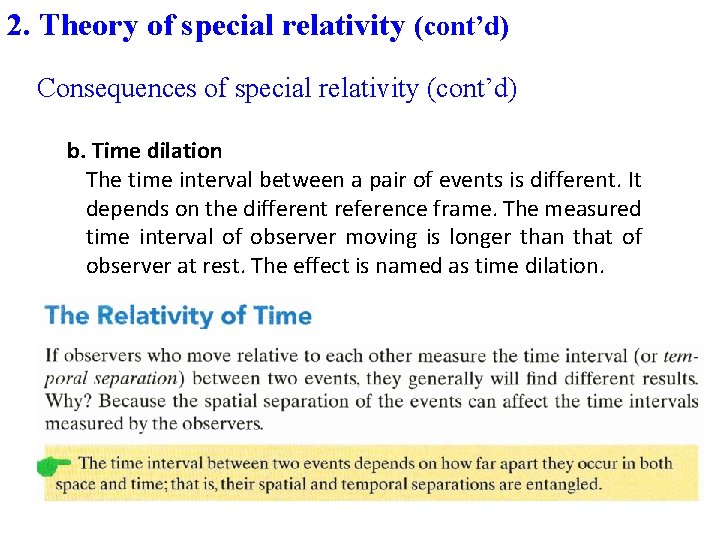
2. Theory of special relativity (cont’d) Consequences of special relativity (cont’d) b. Time dilation The time interval between a pair of events is different. It depends on the different reference frame. The measured time interval of observer moving is longer than that of observer at rest. The effect is named as time dilation.
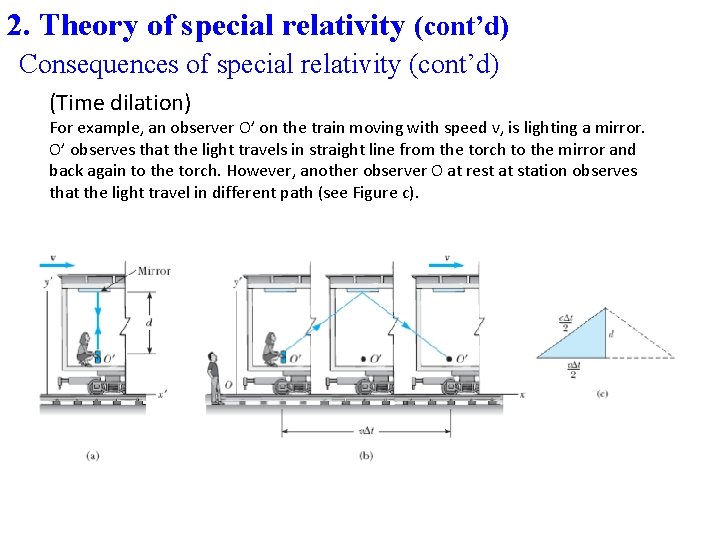
2. Theory of special relativity (cont’d) Consequences of special relativity (cont’d) (Time dilation) For example, an observer O’ on the train moving with speed v, is lighting a mirror. O’ observes that the light travels in straight line from the torch to the mirror and back again to the torch. However, another observer O at rest at station observes that the light travel in different path (see Figure c).
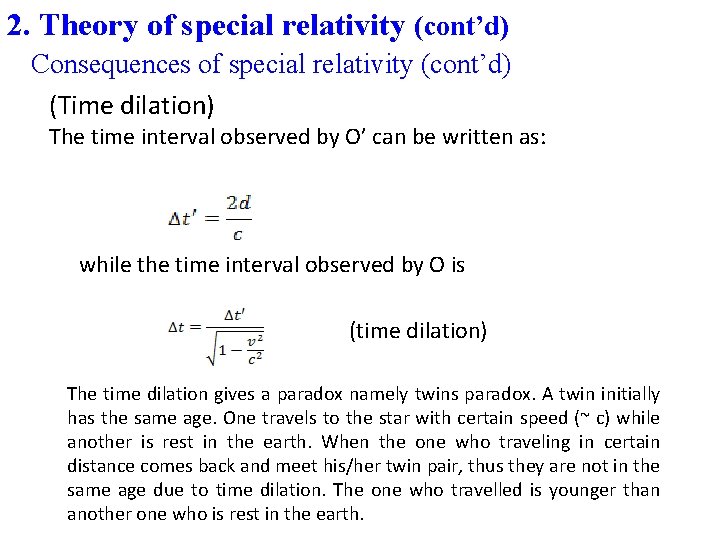
2. Theory of special relativity (cont’d) Consequences of special relativity (cont’d) (Time dilation) The time interval observed by O’ can be written as: while the time interval observed by O is (time dilation) The time dilation gives a paradox namely twins paradox. A twin initially has the same age. One travels to the star with certain speed (~ c) while another is rest in the earth. When the one who traveling in certain distance comes back and meet his/her twin pair, thus they are not in the same age due to time dilation. The one who travelled is younger than another one who is rest in the earth.
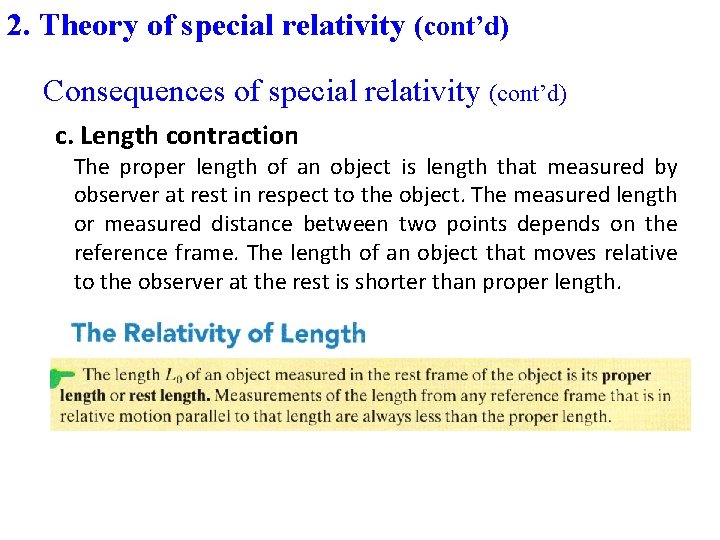
2. Theory of special relativity (cont’d) Consequences of special relativity (cont’d) c. Length contraction The proper length of an object is length that measured by observer at rest in respect to the object. The measured length or measured distance between two points depends on the reference frame. The length of an object that moves relative to the observer at the rest is shorter than proper length.
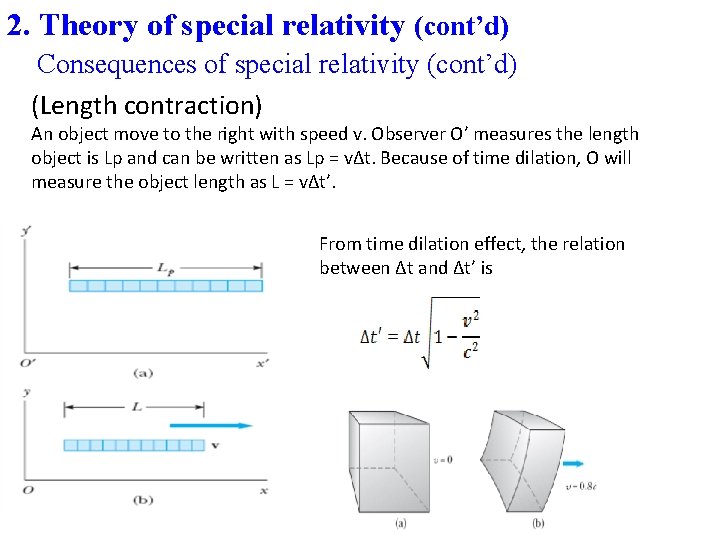
2. Theory of special relativity (cont’d) Consequences of special relativity (cont’d) (Length contraction) An object move to the right with speed v. Observer O’ measures the length object is Lp and can be written as Lp = vΔt. Because of time dilation, O will measure the object length as L = vΔt’. From time dilation effect, the relation between Δt and Δt’ is
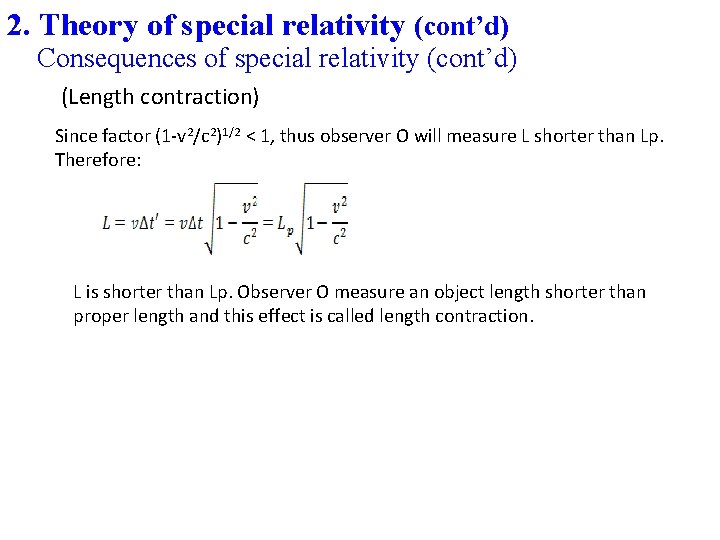
2. Theory of special relativity (cont’d) Consequences of special relativity (cont’d) (Length contraction) Since factor (1 -v 2/c 2)1/2 < 1, thus observer O will measure L shorter than Lp. Therefore: L is shorter than Lp. Observer O measure an object length shorter than proper length and this effect is called length contraction.
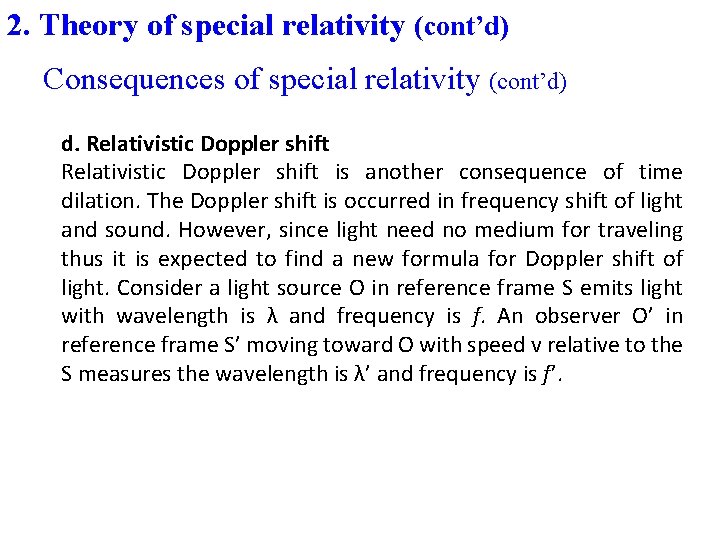
2. Theory of special relativity (cont’d) Consequences of special relativity (cont’d) d. Relativistic Doppler shift is another consequence of time dilation. The Doppler shift is occurred in frequency shift of light and sound. However, since light need no medium for traveling thus it is expected to find a new formula for Doppler shift of light. Consider a light source O in reference frame S emits light with wavelength is λ and frequency is f. An observer O’ in reference frame S’ moving toward O with speed v relative to the S measures the wavelength is λ’ and frequency is f’.
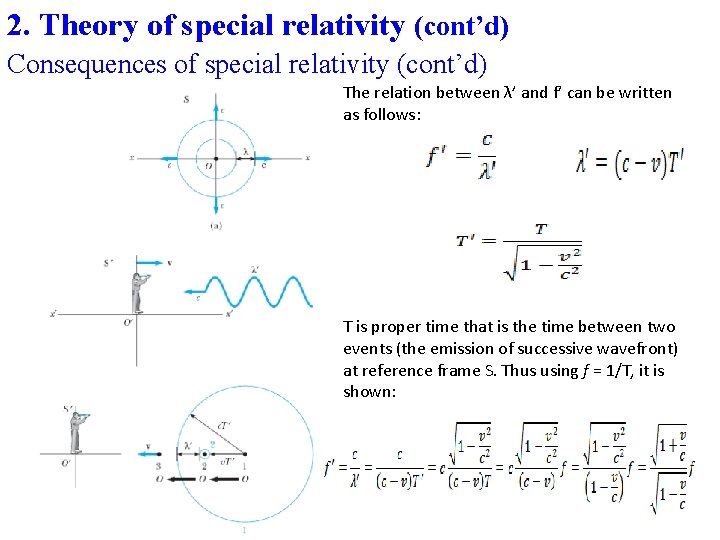
2. Theory of special relativity (cont’d) Consequences of special relativity (cont’d) The relation between λ’ and f’ can be written as follows: T is proper time that is the time between two events (the emission of successive wavefront) at reference frame S. Thus using f = 1/T, it is shown:
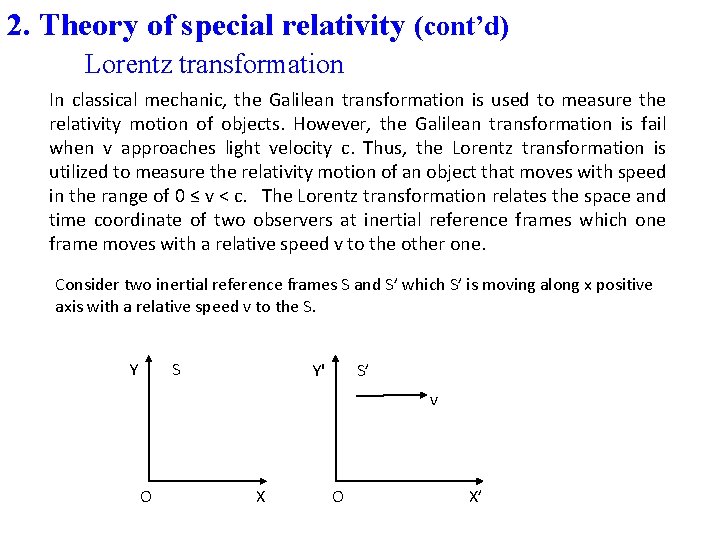
2. Theory of special relativity (cont’d) Lorentz transformation In classical mechanic, the Galilean transformation is used to measure the relativity motion of objects. However, the Galilean transformation is fail when v approaches light velocity c. Thus, the Lorentz transformation is utilized to measure the relativity motion of an object that moves with speed in the range of 0 ≤ v < c. The Lorentz transformation relates the space and time coordinate of two observers at inertial reference frames which one frame moves with a relative speed v to the other one. Consider two inertial reference frames S and S’ which S’ is moving along x positive axis with a relative speed v to the S. Y S Y' S’ v O X’
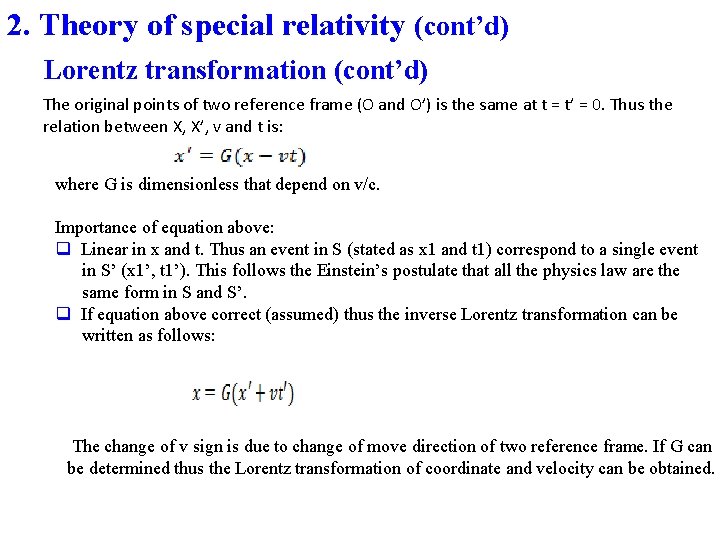
2. Theory of special relativity (cont’d) Lorentz transformation (cont’d) The original points of two reference frame (O and O’) is the same at t = t’ = 0. Thus the relation between X, X’, v and t is: where G is dimensionless that depend on v/c. Importance of equation above: q Linear in x and t. Thus an event in S (stated as x 1 and t 1) correspond to a single event in S’ (x 1’, t 1’). This follows the Einstein’s postulate that all the physics law are the same form in S and S’. q If equation above correct (assumed) thus the inverse Lorentz transformation can be written as follows: The change of v sign is due to change of move direction of two reference frame. If G can be determined thus the Lorentz transformation of coordinate and velocity can be obtained.
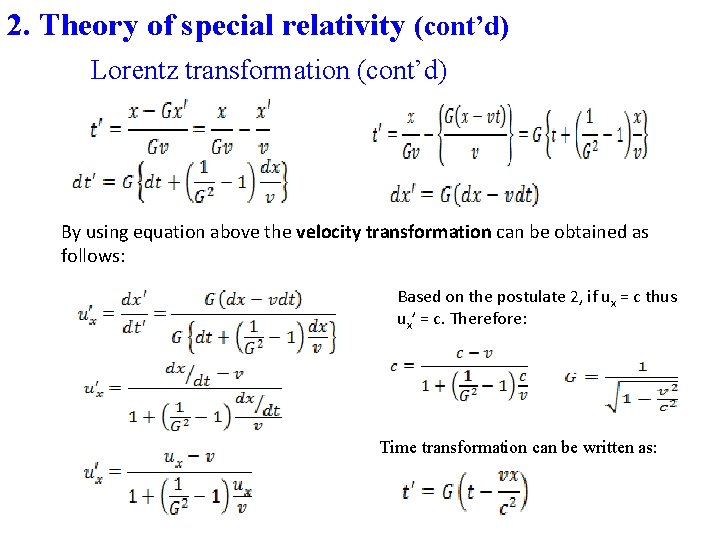
2. Theory of special relativity (cont’d) Lorentz transformation (cont’d) By using equation above the velocity transformation can be obtained as follows: Based on the postulate 2, if ux = c thus ux’ = c. Therefore: Time transformation can be written as:
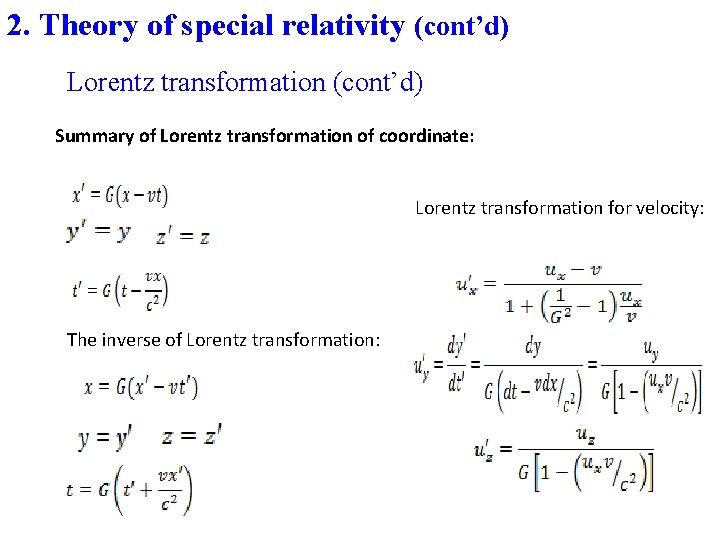
2. Theory of special relativity (cont’d) Lorentz transformation (cont’d) Summary of Lorentz transformation of coordinate: Lorentz transformation for velocity: The inverse of Lorentz transformation:
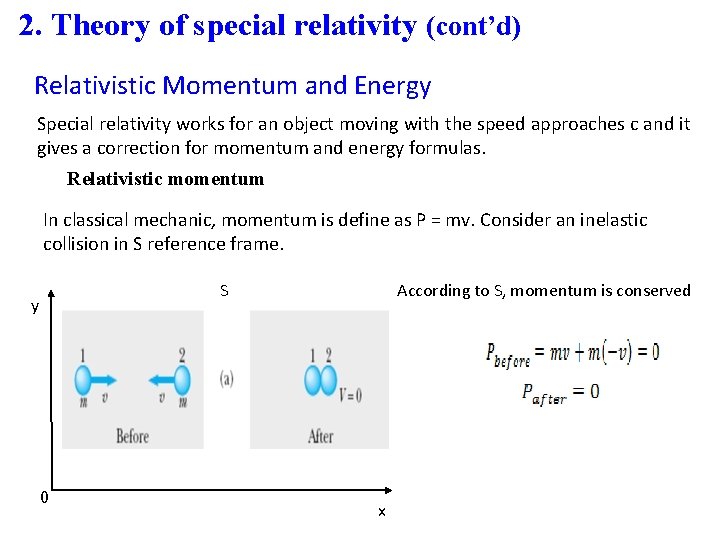
2. Theory of special relativity (cont’d) Relativistic Momentum and Energy Special relativity works for an object moving with the speed approaches c and it gives a correction for momentum and energy formulas. Relativistic momentum In classical mechanic, momentum is define as P = mv. Consider an inelastic collision in S reference frame. S y 0 According to S, momentum is conserved x
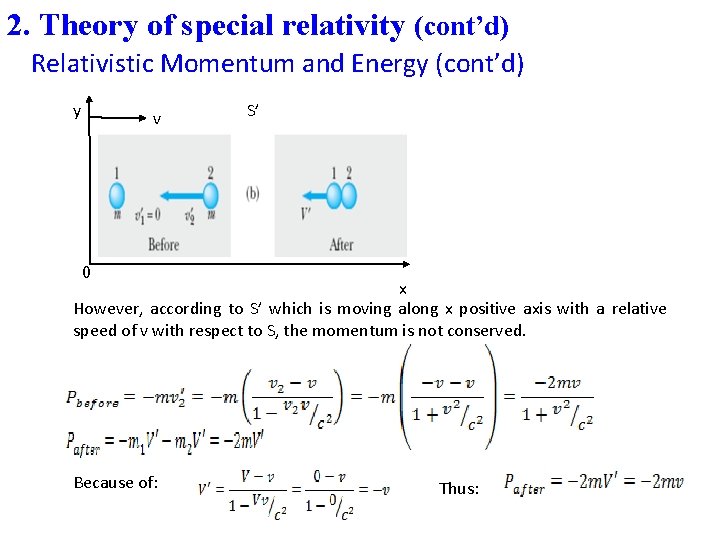
2. Theory of special relativity (cont’d) Relativistic Momentum and Energy (cont’d) y v S’ 0 x However, according to S’ which is moving along x positive axis with a relative speed of v with respect to S, the momentum is not conserved. Because of: Thus:
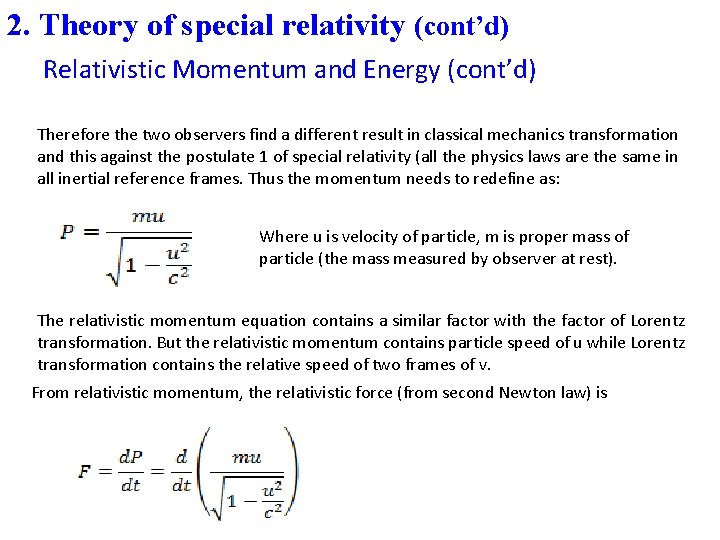
2. Theory of special relativity (cont’d) Relativistic Momentum and Energy (cont’d) Therefore the two observers find a different result in classical mechanics transformation and this against the postulate 1 of special relativity (all the physics laws are the same in all inertial reference frames. Thus the momentum needs to redefine as: Where u is velocity of particle, m is proper mass of particle (the mass measured by observer at rest). The relativistic momentum equation contains a similar factor with the factor of Lorentz transformation. But the relativistic momentum contains particle speed of u while Lorentz transformation contains the relative speed of two frames of v. From relativistic momentum, the relativistic force (from second Newton law) is
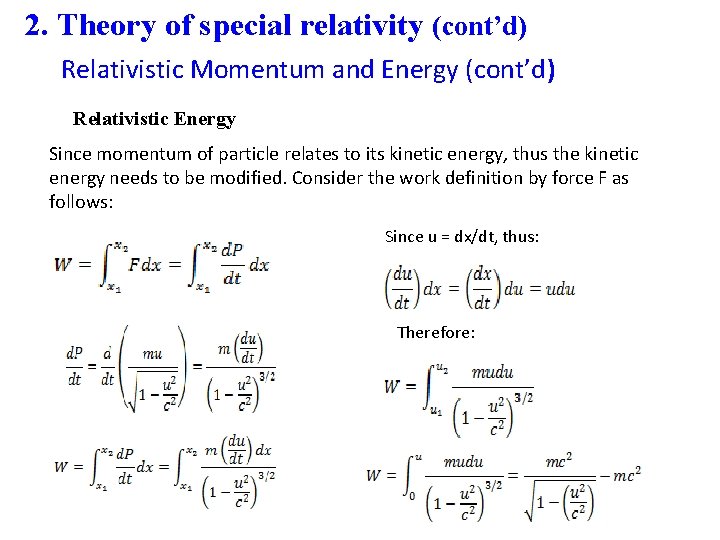
2. Theory of special relativity (cont’d) Relativistic Momentum and Energy (cont’d) Relativistic Energy Since momentum of particle relates to its kinetic energy, thus the kinetic energy needs to be modified. Consider the work definition by force F as follows: Since u = dx/dt, thus: Therefore:
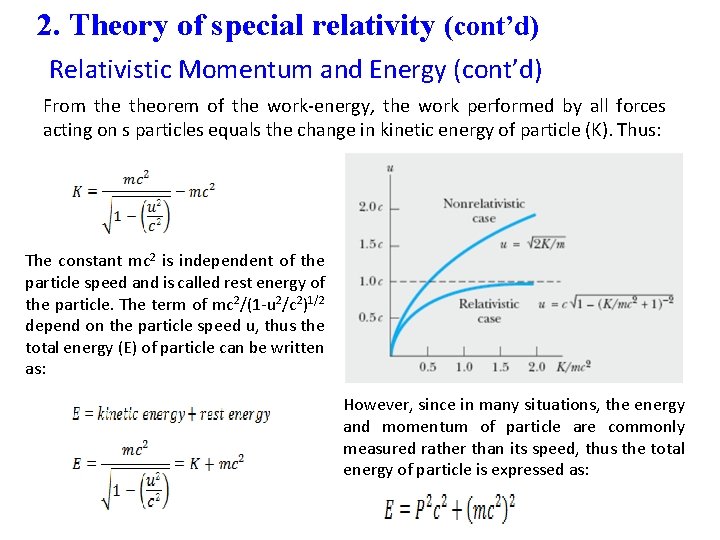
2. Theory of special relativity (cont’d) Relativistic Momentum and Energy (cont’d) From theorem of the work-energy, the work performed by all forces acting on s particles equals the change in kinetic energy of particle (K). Thus: The constant mc 2 is independent of the particle speed and is called rest energy of the particle. The term of mc 2/(1 -u 2/c 2)1/2 depend on the particle speed u, thus the total energy (E) of particle can be written as: However, since in many situations, the energy and momentum of particle are commonly measured rather than its speed, thus the total energy of particle is expressed as:
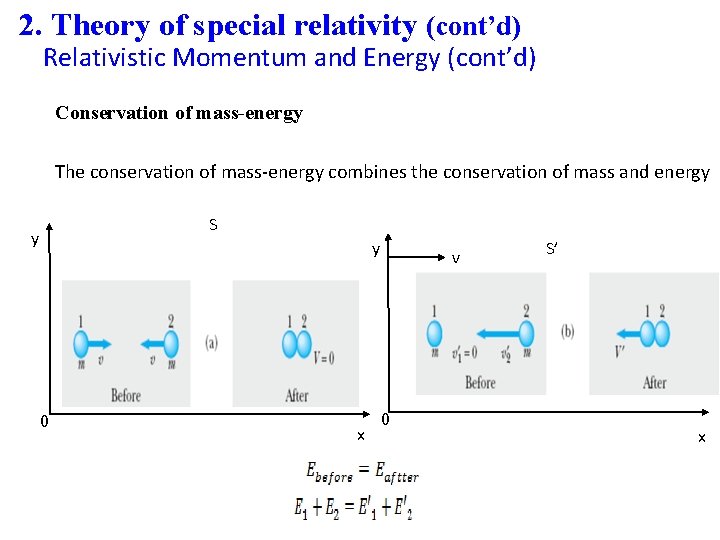
2. Theory of special relativity (cont’d) Relativistic Momentum and Energy (cont’d) Conservation of mass-energy The conservation of mass-energy combines the conservation of mass and energy S y y 0 x v 0 S’ x
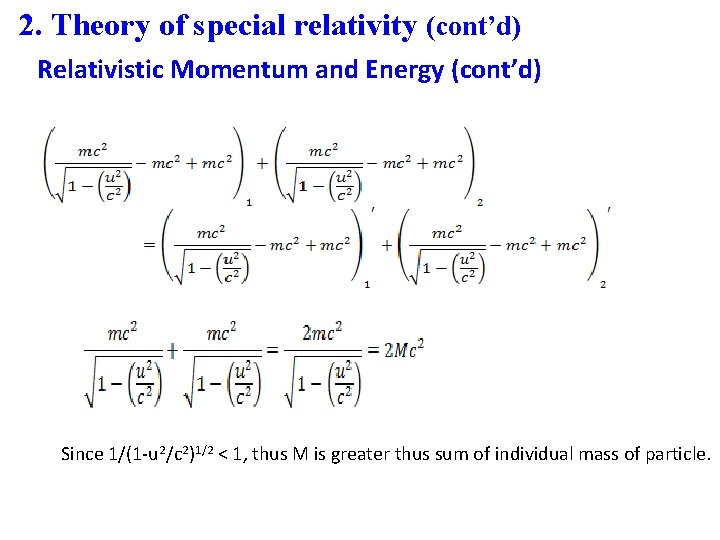
2. Theory of special relativity (cont’d) Relativistic Momentum and Energy (cont’d) Since 1/(1 -u 2/c 2)1/2 < 1, thus M is greater thus sum of individual mass of particle.
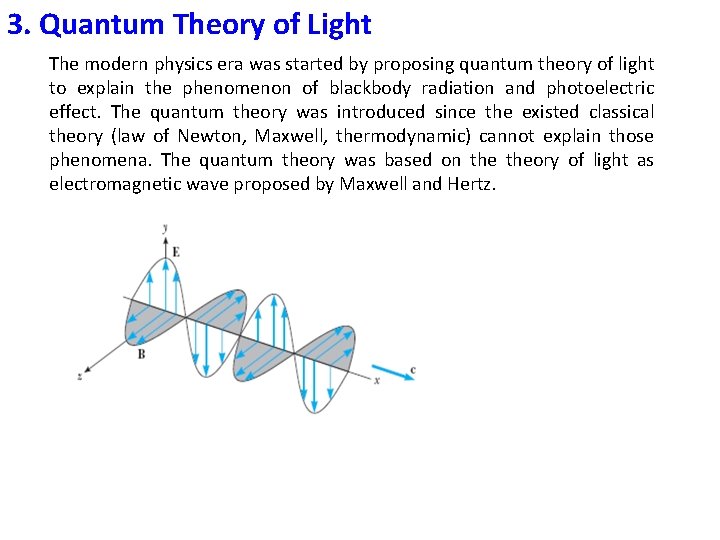
3. Quantum Theory of Light The modern physics era was started by proposing quantum theory of light to explain the phenomenon of blackbody radiation and photoelectric effect. The quantum theory was introduced since the existed classical theory (law of Newton, Maxwell, thermodynamic) cannot explain those phenomena. The quantum theory was based on theory of light as electromagnetic wave proposed by Maxwell and Hertz.
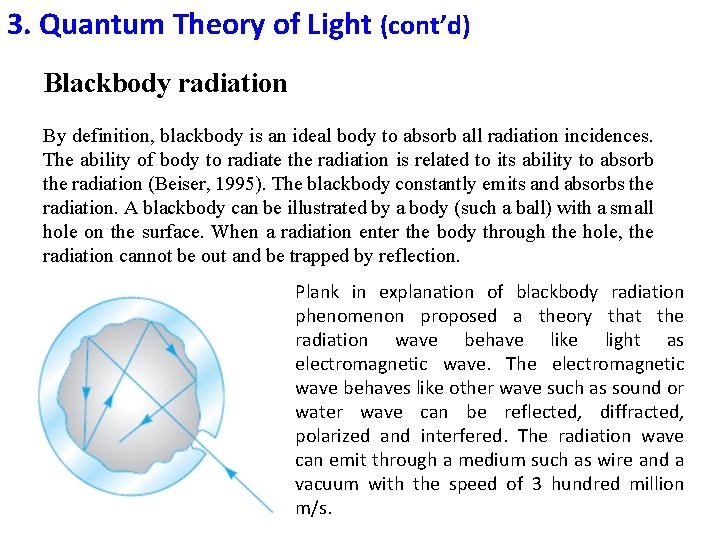
3. Quantum Theory of Light (cont’d) Blackbody radiation By definition, blackbody is an ideal body to absorb all radiation incidences. The ability of body to radiate the radiation is related to its ability to absorb the radiation (Beiser, 1995). The blackbody constantly emits and absorbs the radiation. A blackbody can be illustrated by a body (such a ball) with a small hole on the surface. When a radiation enter the body through the hole, the radiation cannot be out and be trapped by reflection. Plank in explanation of blackbody radiation phenomenon proposed a theory that the radiation wave behave like light as electromagnetic wave. The electromagnetic wave behaves like other wave such as sound or water wave can be reflected, diffracted, polarized and interfered. The radiation wave can emit through a medium such as wire and a vacuum with the speed of 3 hundred million m/s.
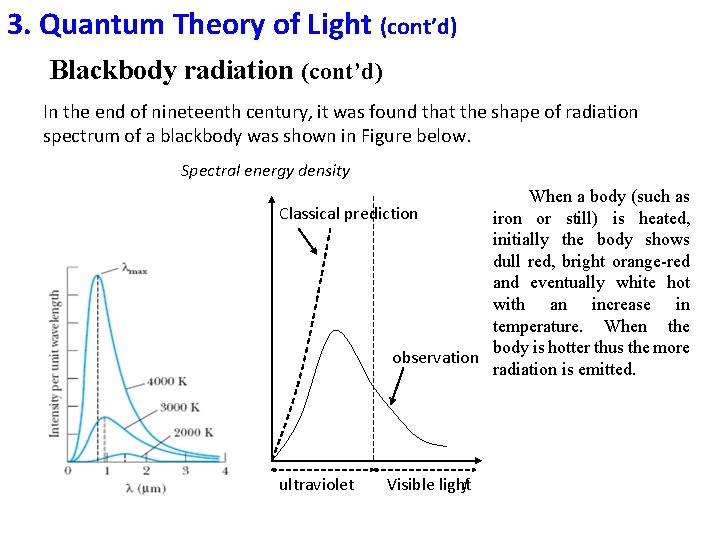
3. Quantum Theory of Light (cont’d) Blackbody radiation (cont’d) In the end of nineteenth century, it was found that the shape of radiation spectrum of a blackbody was shown in Figure below. Spectral energy density When a body (such as Classical prediction iron or still) is heated, initially the body shows dull red, bright orange-red and eventually white hot with an increase in temperature. When the body is hotter thus the more observation radiation is emitted. ultraviolet Visible lightf
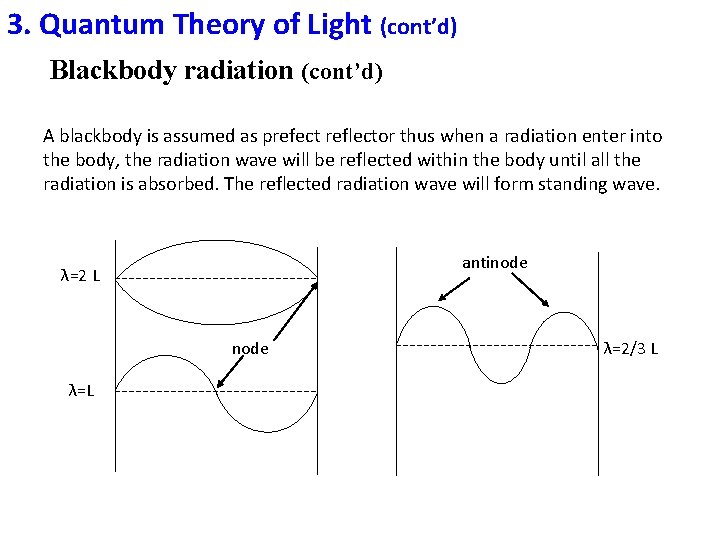
3. Quantum Theory of Light (cont’d) Blackbody radiation (cont’d) A blackbody is assumed as prefect reflector thus when a radiation enter into the body, the radiation wave will be reflected within the body until all the radiation is absorbed. The reflected radiation wave will form standing wave. antinode λ=2 L node λ=L λ=2/3 L
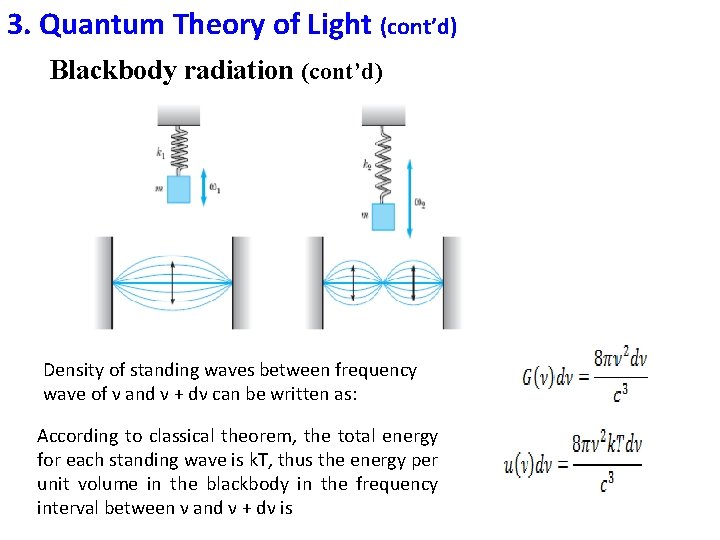
3. Quantum Theory of Light (cont’d) Blackbody radiation (cont’d) Density of standing waves between frequency wave of ν and ν + dν can be written as: According to classical theorem, the total energy for each standing wave is k. T, thus the energy per unit volume in the blackbody in the frequency interval between ν and ν + dν is
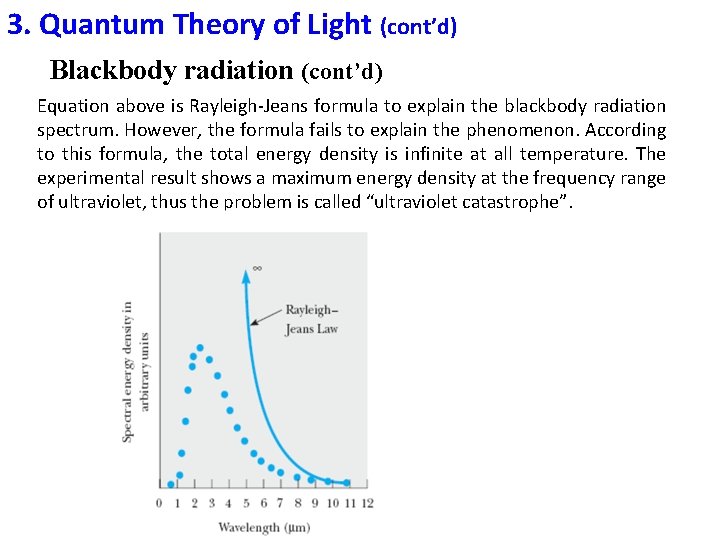
3. Quantum Theory of Light (cont’d) Blackbody radiation (cont’d) Equation above is Rayleigh-Jeans formula to explain the blackbody radiation spectrum. However, the formula fails to explain the phenomenon. According to this formula, the total energy density is infinite at all temperature. The experimental result shows a maximum energy density at the frequency range of ultraviolet, thus the problem is called “ultraviolet catastrophe”.
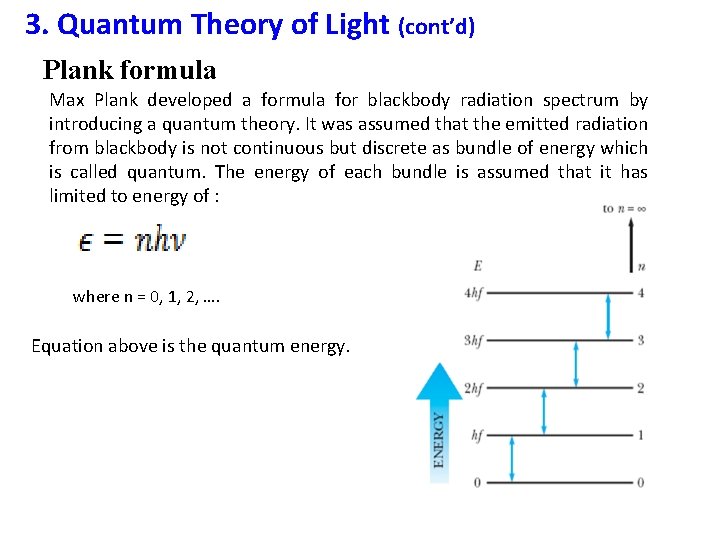
3. Quantum Theory of Light (cont’d) Plank formula Max Plank developed a formula for blackbody radiation spectrum by introducing a quantum theory. It was assumed that the emitted radiation from blackbody is not continuous but discrete as bundle of energy which is called quantum. The energy of each bundle is assumed that it has limited to energy of : where n = 0, 1, 2, …. Equation above is the quantum energy.
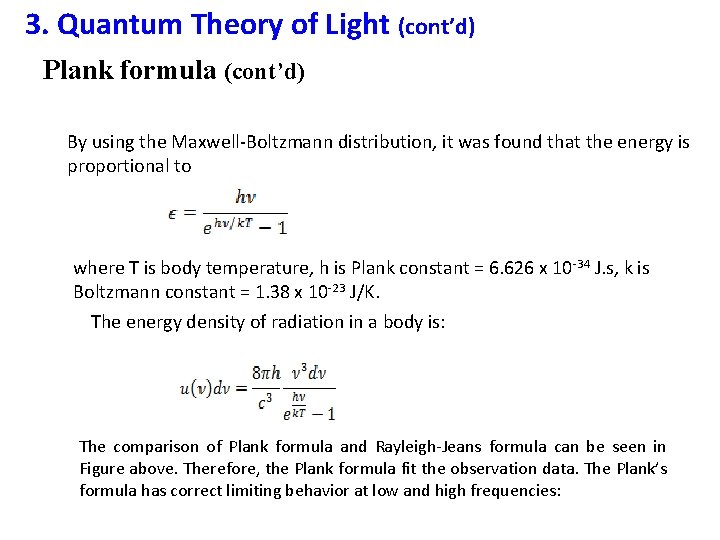
3. Quantum Theory of Light (cont’d) Plank formula (cont’d) By using the Maxwell-Boltzmann distribution, it was found that the energy is proportional to where T is body temperature, h is Plank constant = 6. 626 x 10 -34 J. s, k is Boltzmann constant = 1. 38 x 10 -23 J/K. The energy density of radiation in a body is: The comparison of Plank formula and Rayleigh-Jeans formula can be seen in Figure above. Therefore, the Plank formula fit the observation data. The Plank’s formula has correct limiting behavior at low and high frequencies:
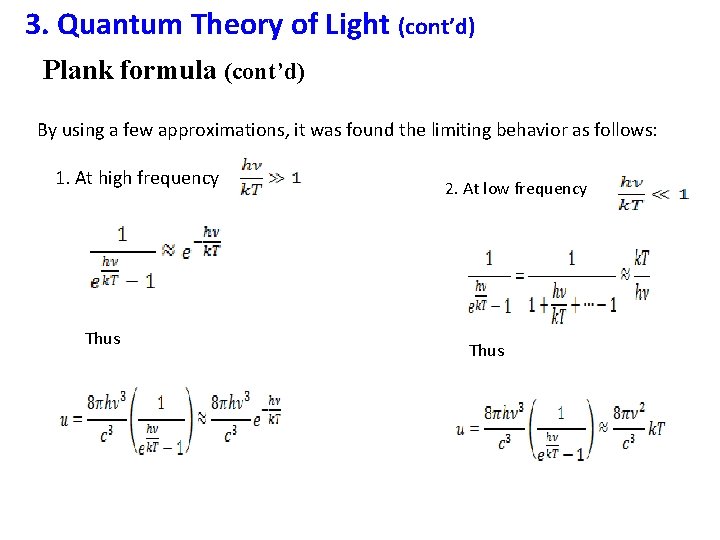
3. Quantum Theory of Light (cont’d) Plank formula (cont’d) By using a few approximations, it was found the limiting behavior as follows: 1. At high frequency Thus 2. At low frequency Thus
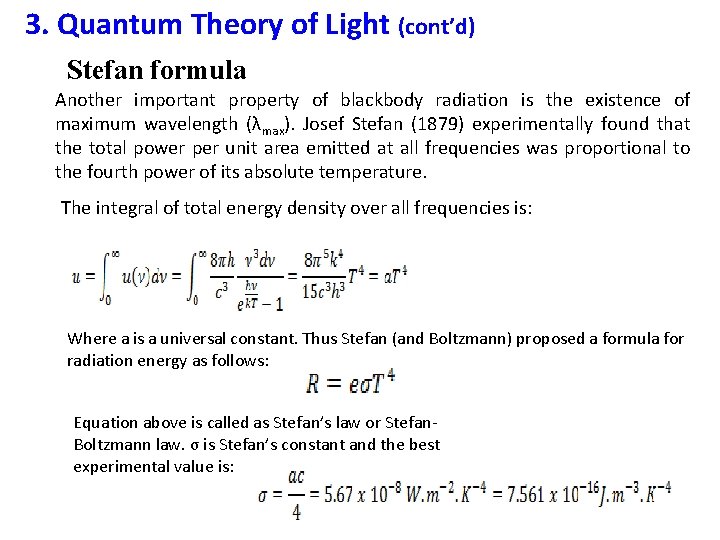
3. Quantum Theory of Light (cont’d) Stefan formula Another important property of blackbody radiation is the existence of maximum wavelength (λmax). Josef Stefan (1879) experimentally found that the total power per unit area emitted at all frequencies was proportional to the fourth power of its absolute temperature. The integral of total energy density over all frequencies is: Where a is a universal constant. Thus Stefan (and Boltzmann) proposed a formula for radiation energy as follows: Equation above is called as Stefan’s law or Stefan. Boltzmann law. σ is Stefan’s constant and the best experimental value is:
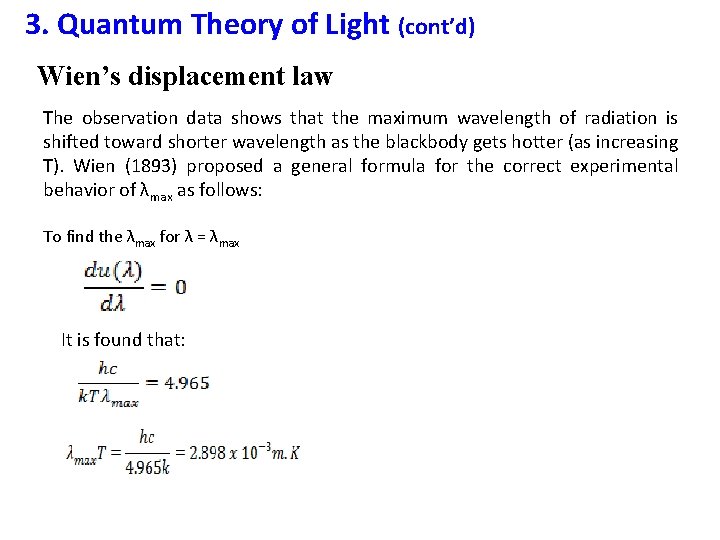
3. Quantum Theory of Light (cont’d) Wien’s displacement law The observation data shows that the maximum wavelength of radiation is shifted toward shorter wavelength as the blackbody gets hotter (as increasing T). Wien (1893) proposed a general formula for the correct experimental behavior of λmax as follows: To find the λmax for λ = λmax It is found that:
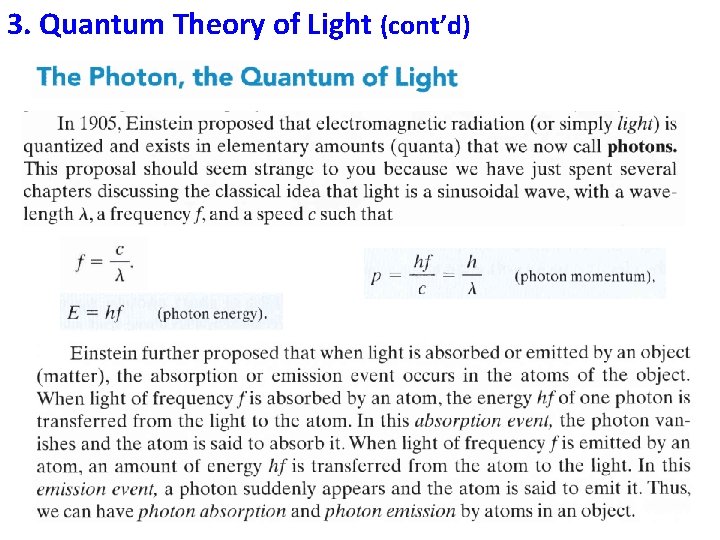
3. Quantum Theory of Light (cont’d)
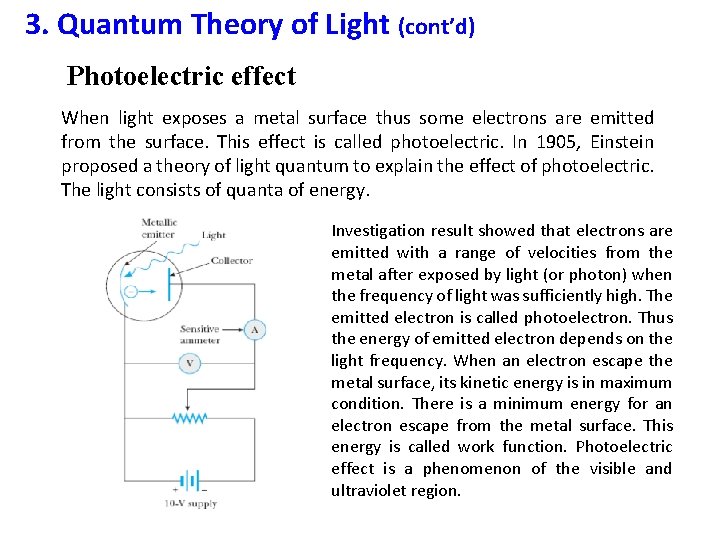
3. Quantum Theory of Light (cont’d) Photoelectric effect When light exposes a metal surface thus some electrons are emitted from the surface. This effect is called photoelectric. In 1905, Einstein proposed a theory of light quantum to explain the effect of photoelectric. The light consists of quanta of energy. Investigation result showed that electrons are emitted with a range of velocities from the metal after exposed by light (or photon) when the frequency of light was sufficiently high. The emitted electron is called photoelectron. Thus the energy of emitted electron depends on the light frequency. When an electron escape the metal surface, its kinetic energy is in maximum condition. There is a minimum energy for an electron escape from the metal surface. This energy is called work function. Photoelectric effect is a phenomenon of the visible and ultraviolet region.
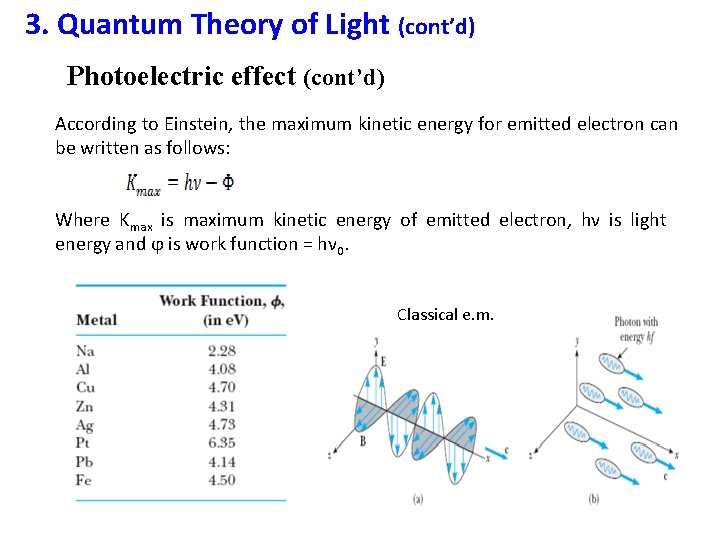
3. Quantum Theory of Light (cont’d) Photoelectric effect (cont’d) According to Einstein, the maximum kinetic energy for emitted electron can be written as follows: Where Kmax is maximum kinetic energy of emitted electron, hν is light energy and ϕ is work function = hν 0. Classical e. m.
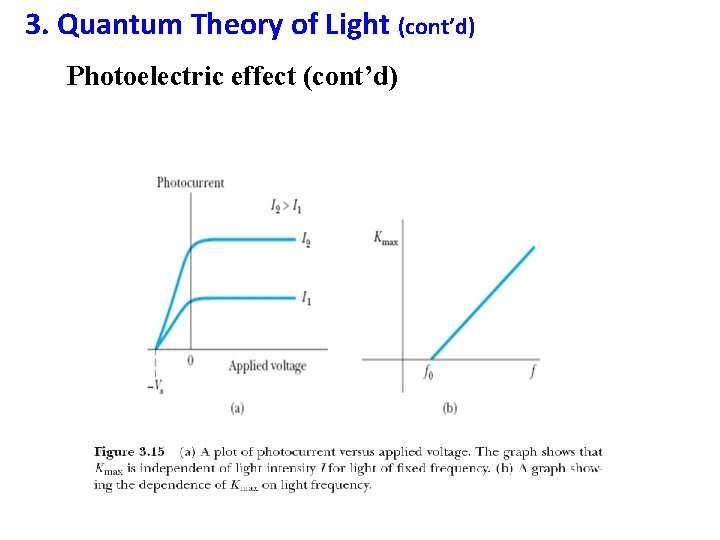
3. Quantum Theory of Light (cont’d) Photoelectric effect (cont’d)
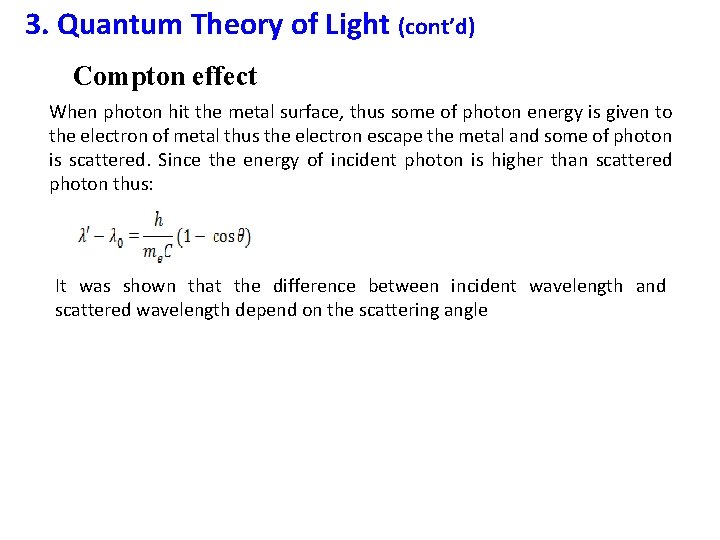
3. Quantum Theory of Light (cont’d) Compton effect When photon hit the metal surface, thus some of photon energy is given to the electron of metal thus the electron escape the metal and some of photon is scattered. Since the energy of incident photon is higher than scattered photon thus: It was shown that the difference between incident wavelength and scattered wavelength depend on the scattering angle
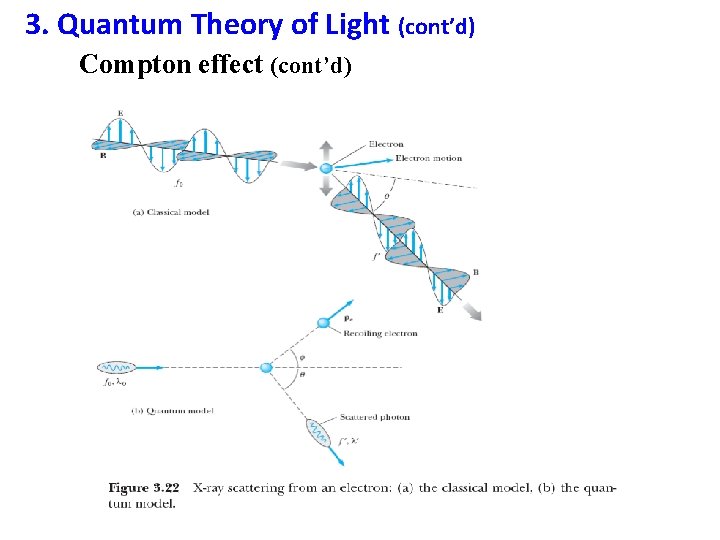
3. Quantum Theory of Light (cont’d) Compton effect (cont’d)
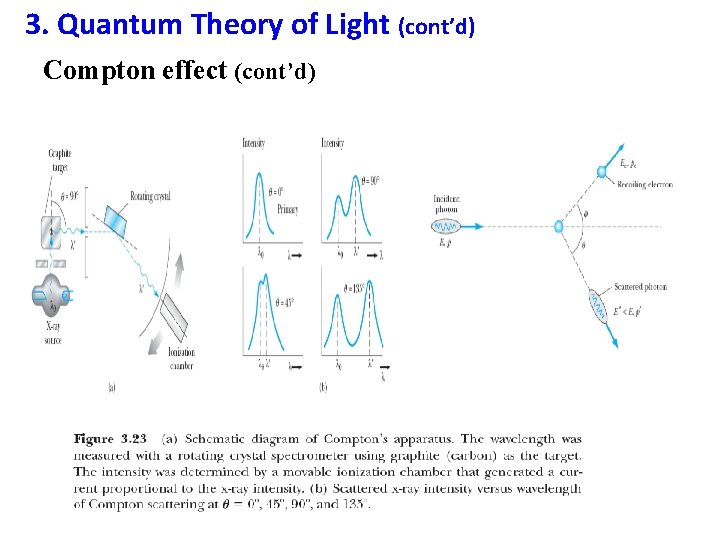
3. Quantum Theory of Light (cont’d) Compton effect (cont’d)
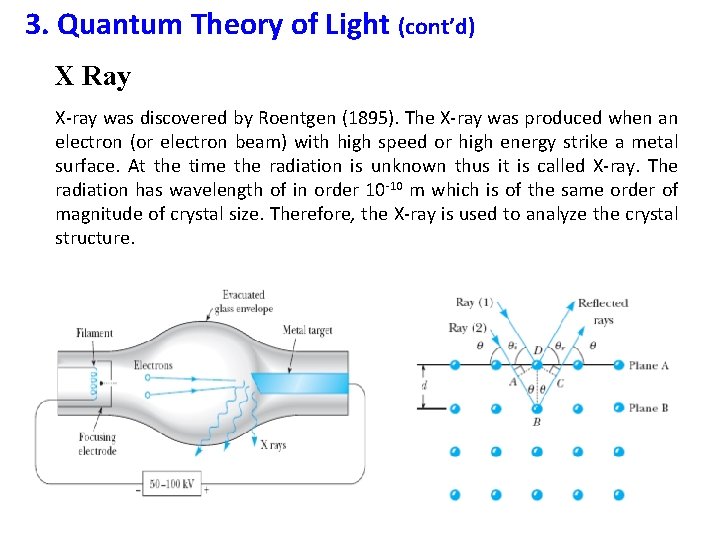
3. Quantum Theory of Light (cont’d) X Ray X-ray was discovered by Roentgen (1895). The X-ray was produced when an electron (or electron beam) with high speed or high energy strike a metal surface. At the time the radiation is unknown thus it is called X-ray. The radiation has wavelength of in order 10 -10 m which is of the same order of magnitude of crystal size. Therefore, the X-ray is used to analyze the crystal structure.
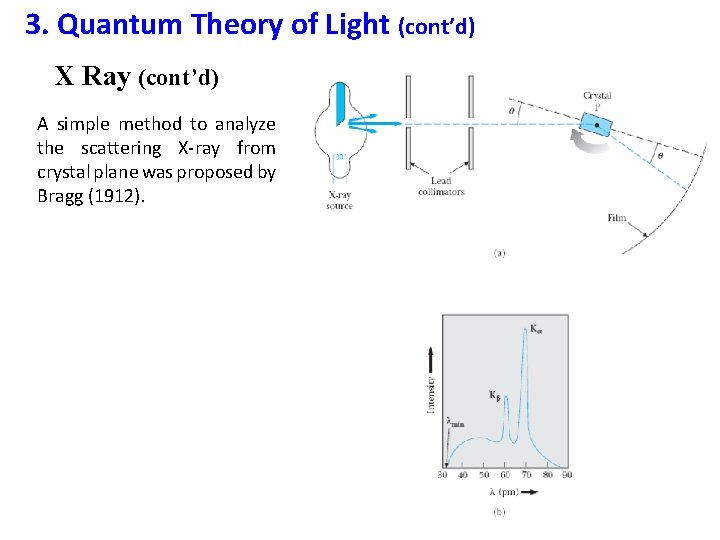
3. Quantum Theory of Light (cont’d) X Ray (cont’d) A simple method to analyze the scattering X-ray from crystal plane was proposed by Bragg (1912).
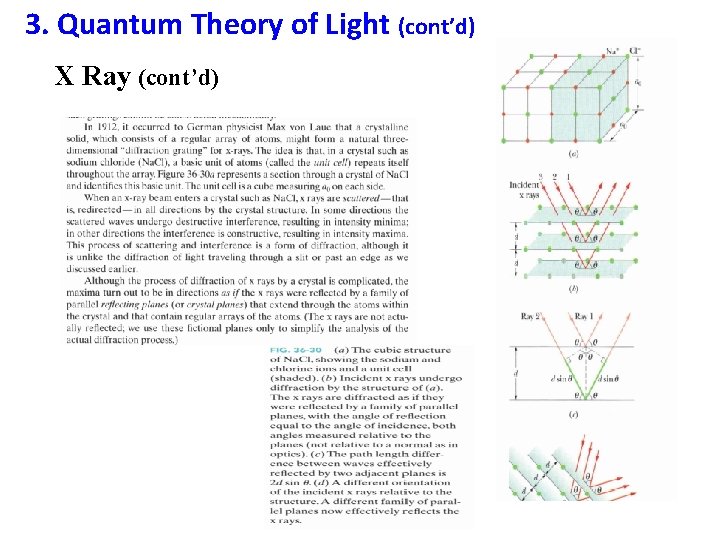
3. Quantum Theory of Light (cont’d) X Ray (cont’d)
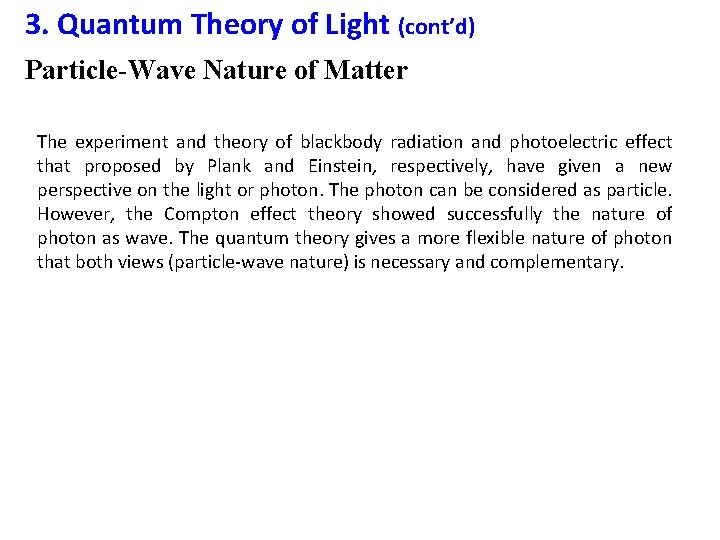
3. Quantum Theory of Light (cont’d) Particle-Wave Nature of Matter The experiment and theory of blackbody radiation and photoelectric effect that proposed by Plank and Einstein, respectively, have given a new perspective on the light or photon. The photon can be considered as particle. However, the Compton effect theory showed successfully the nature of photon as wave. The quantum theory gives a more flexible nature of photon that both views (particle-wave nature) is necessary and complementary.
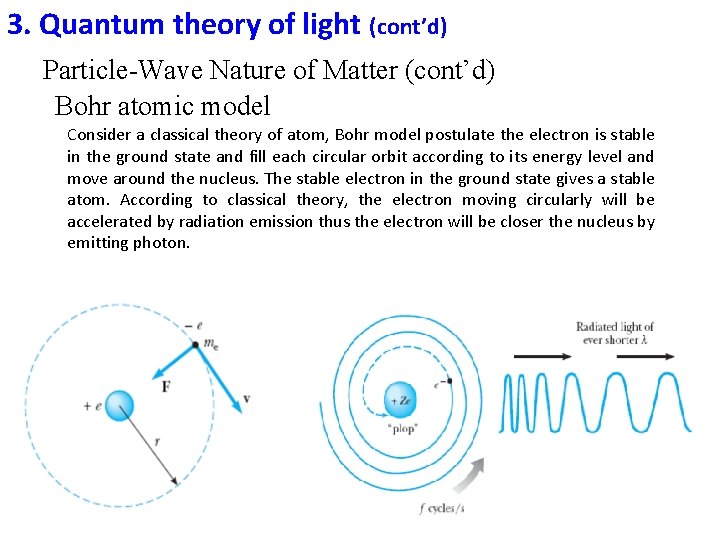
3. Quantum theory of light (cont’d) Particle-Wave Nature of Matter (cont’d) Bohr atomic model Consider a classical theory of atom, Bohr model postulate the electron is stable in the ground state and fill each circular orbit according to its energy level and move around the nucleus. The stable electron in the ground state gives a stable atom. According to classical theory, the electron moving circularly will be accelerated by radiation emission thus the electron will be closer the nucleus by emitting photon.
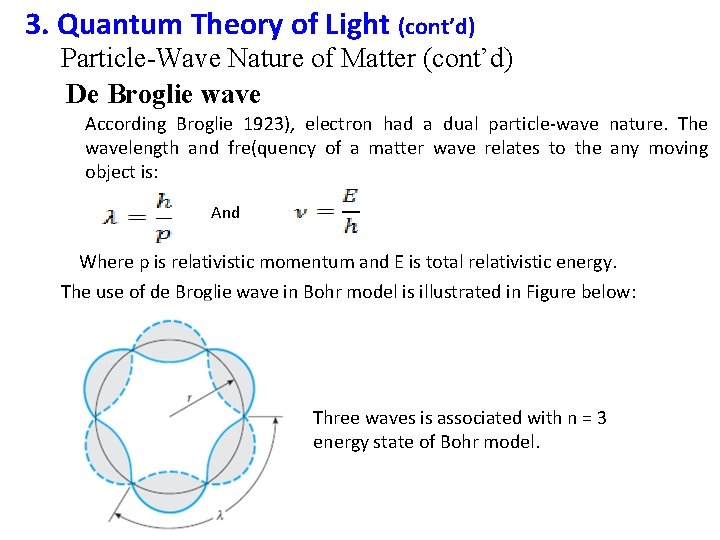
3. Quantum Theory of Light (cont’d) Particle-Wave Nature of Matter (cont’d) De Broglie wave According Broglie 1923), electron had a dual particle-wave nature. The wavelength and fre(quency of a matter wave relates to the any moving object is: And Where p is relativistic momentum and E is total relativistic energy. The use of de Broglie wave in Bohr model is illustrated in Figure below: Three waves is associated with n = 3 energy state of Bohr model.
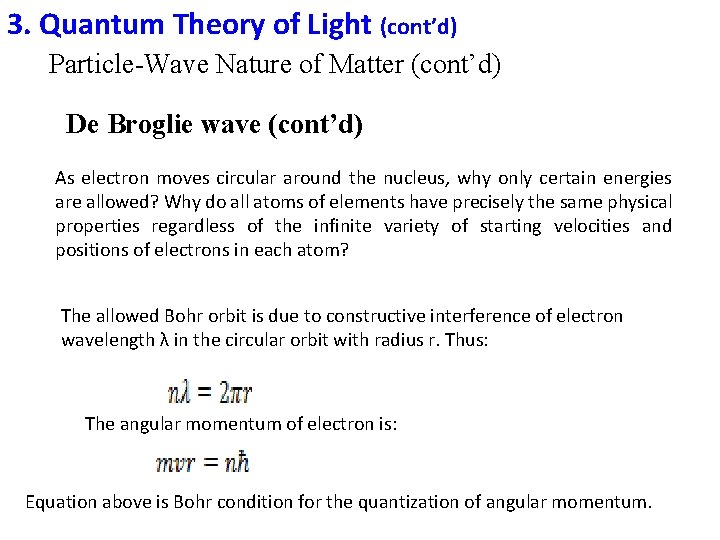
3. Quantum Theory of Light (cont’d) Particle-Wave Nature of Matter (cont’d) De Broglie wave (cont’d) As electron moves circular around the nucleus, why only certain energies are allowed? Why do all atoms of elements have precisely the same physical properties regardless of the infinite variety of starting velocities and positions of electrons in each atom? The allowed Bohr orbit is due to constructive interference of electron wavelength λ in the circular orbit with radius r. Thus: The angular momentum of electron is: Equation above is Bohr condition for the quantization of angular momentum.
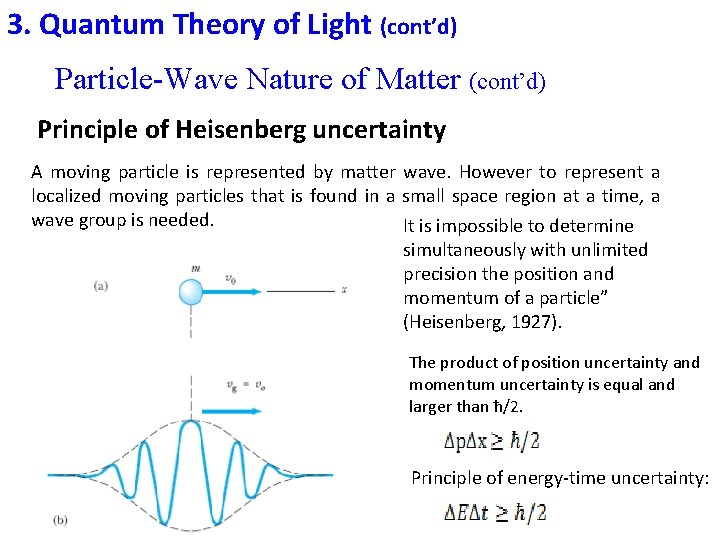
3. Quantum Theory of Light (cont’d) Particle-Wave Nature of Matter (cont’d) Principle of Heisenberg uncertainty A moving particle is represented by matter wave. However to represent a localized moving particles that is found in a small space region at a time, a wave group is needed. It is impossible to determine simultaneously with unlimited precision the position and momentum of a particle” (Heisenberg, 1927). The product of position uncertainty and momentum uncertainty is equal and larger than ħ/2. Principle of energy-time uncertainty:
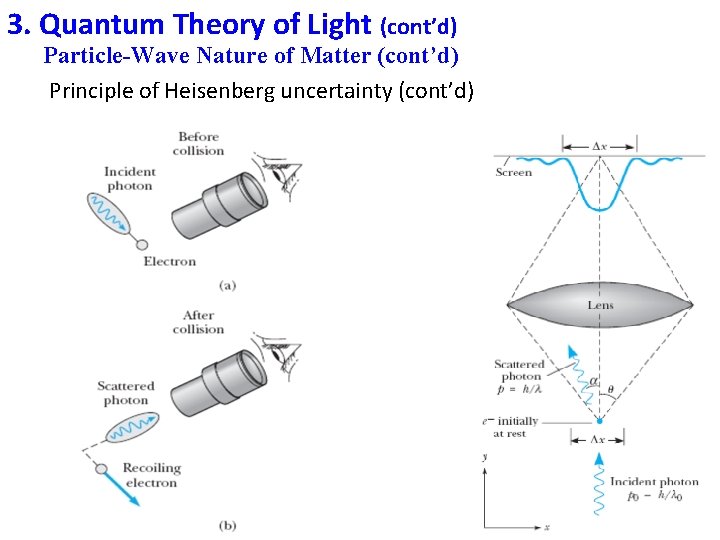
3. Quantum Theory of Light (cont’d) Particle-Wave Nature of Matter (cont’d) Principle of Heisenberg uncertainty (cont’d)
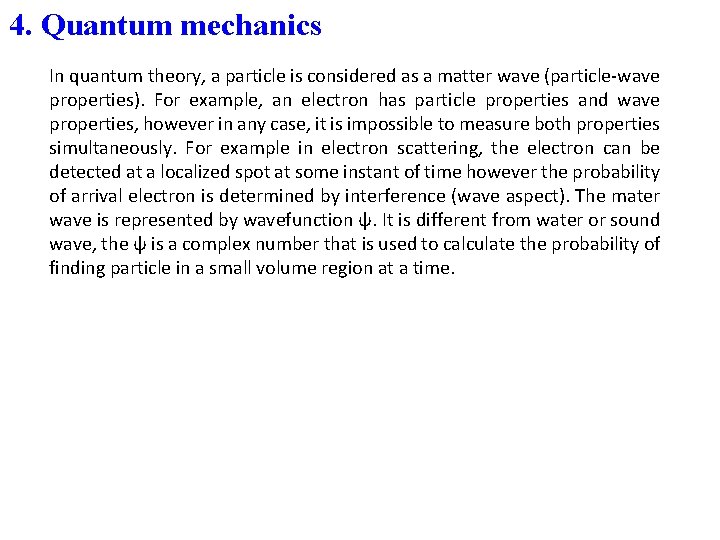
4. Quantum mechanics In quantum theory, a particle is considered as a matter wave (particle-wave properties). For example, an electron has particle properties and wave properties, however in any case, it is impossible to measure both properties simultaneously. For example in electron scattering, the electron can be detected at a localized spot at some instant of time however the probability of arrival electron is determined by interference (wave aspect). The mater wave is represented by wavefunction ψ. It is different from water or sound wave, the ψ is a complex number that is used to calculate the probability of finding particle in a small volume region at a time.
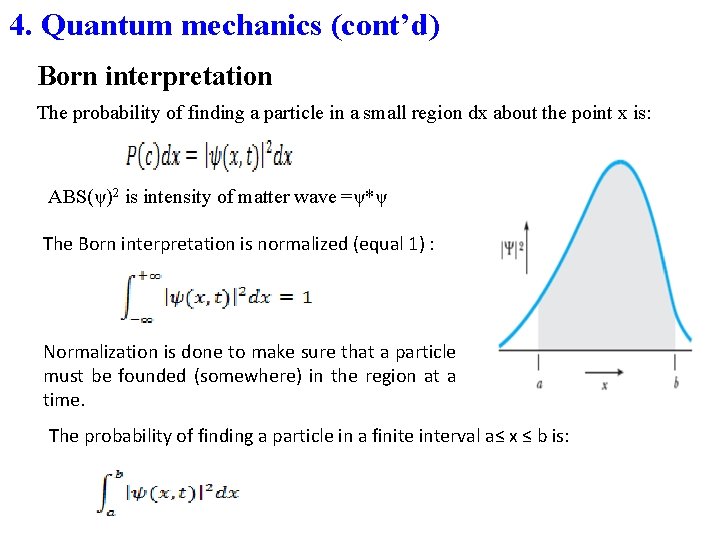
4. Quantum mechanics (cont’d) Born interpretation The probability of finding a particle in a small region dx about the point x is: ABS(ψ)2 is intensity of matter wave =ψ*ψ The Born interpretation is normalized (equal 1) : Normalization is done to make sure that a particle must be founded (somewhere) in the region at a time. The probability of finding a particle in a finite interval a≤ x ≤ b is:
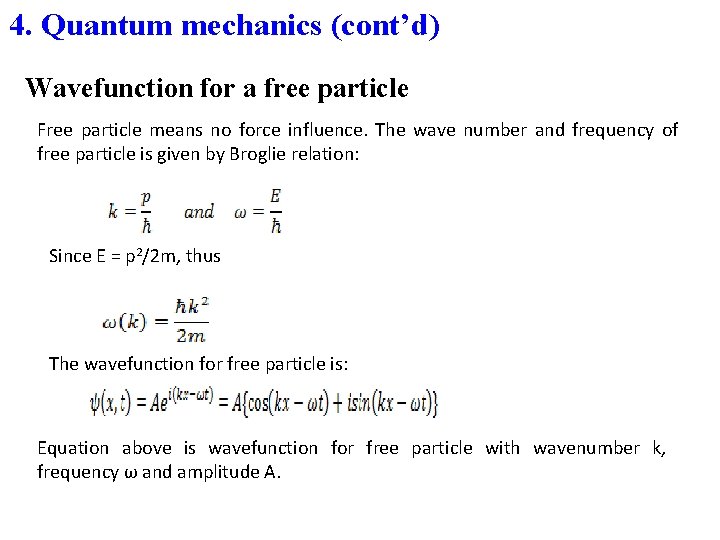
4. Quantum mechanics (cont’d) Wavefunction for a free particle Free particle means no force influence. The wave number and frequency of free particle is given by Broglie relation: Since E = p 2/2 m, thus The wavefunction for free particle is: Equation above is wavefunction for free particle with wavenumber k, frequency ω and amplitude A.
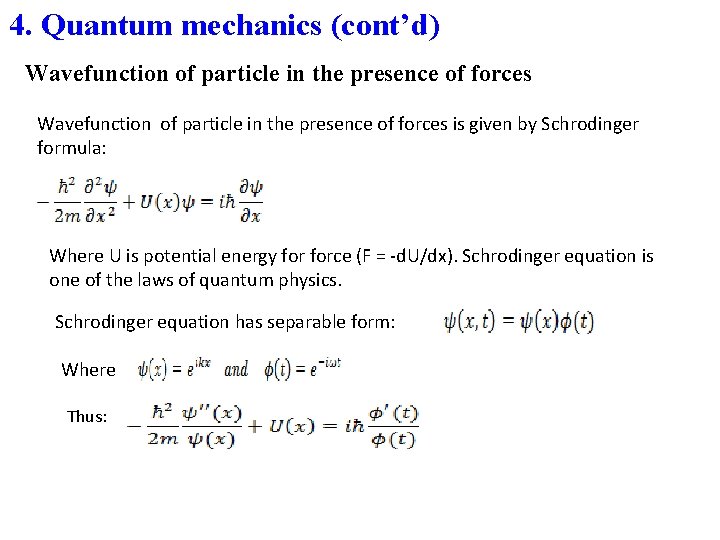
4. Quantum mechanics (cont’d) Wavefunction of particle in the presence of forces is given by Schrodinger formula: Where U is potential energy force (F = -d. U/dx). Schrodinger equation is one of the laws of quantum physics. Schrodinger equation has separable form: Where Thus:
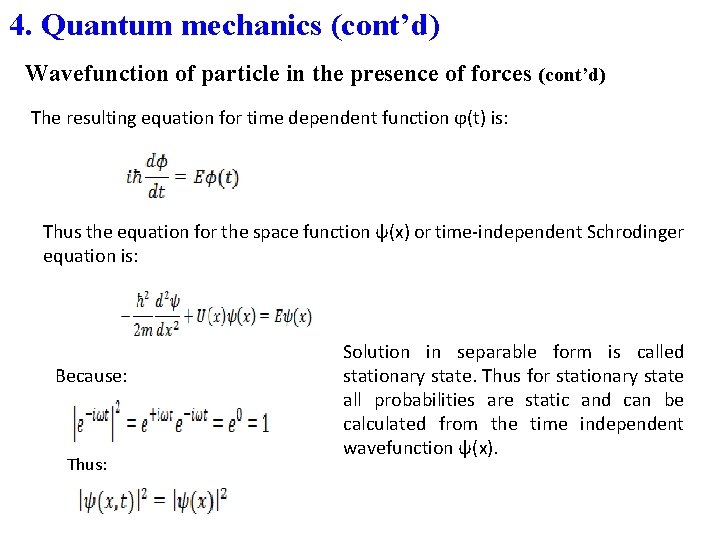
4. Quantum mechanics (cont’d) Wavefunction of particle in the presence of forces (cont’d) The resulting equation for time dependent function ϕ(t) is: Thus the equation for the space function ψ(x) or time-independent Schrodinger equation is: Because: Thus: Solution in separable form is called stationary state. Thus for stationary state all probabilities are static and can be calculated from the time independent wavefunction ψ(x).
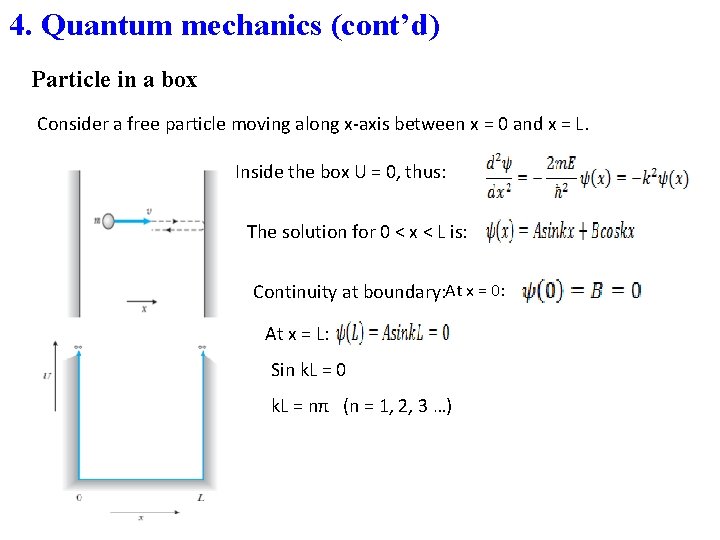
4. Quantum mechanics (cont’d) Particle in a box Consider a free particle moving along x-axis between x = 0 and x = L. Inside the box U = 0, thus: The solution for 0 < x < L is: Continuity at boundary: At x = 0: At x = L: Sin k. L = 0 k. L = nπ (n = 1, 2, 3 …)
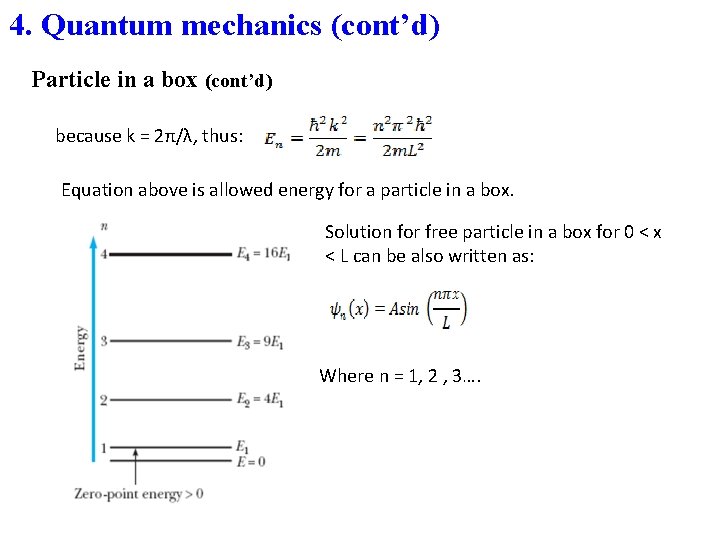
4. Quantum mechanics (cont’d) Particle in a box (cont’d) because k = 2π/λ, thus: Equation above is allowed energy for a particle in a box. Solution for free particle in a box for 0 < x < L can be also written as: Where n = 1, 2 , 3….
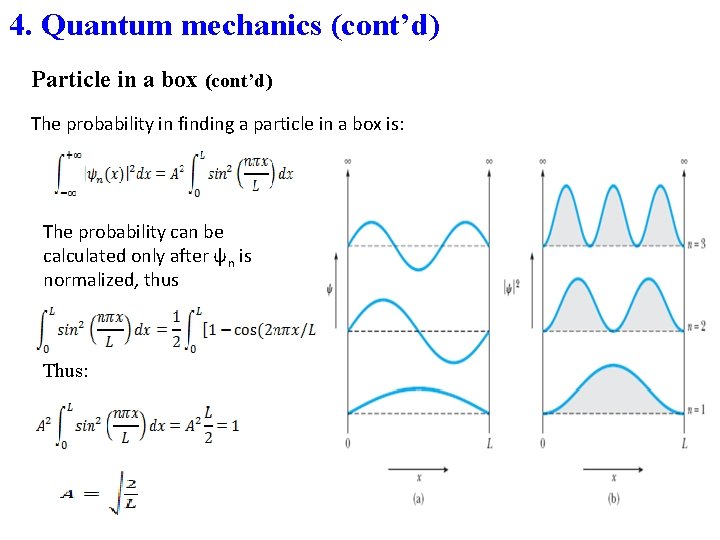
4. Quantum mechanics (cont’d) Particle in a box (cont’d) The probability in finding a particle in a box is: The probability can be calculated only after ψn is normalized, thus Thus:
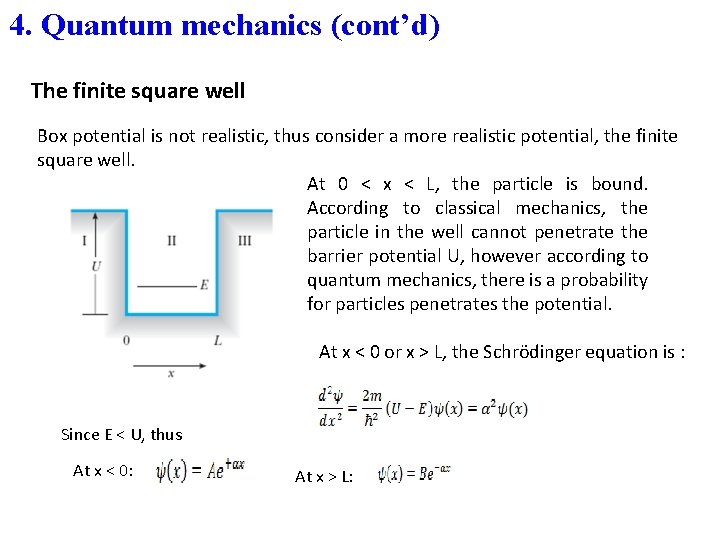
4. Quantum mechanics (cont’d) The finite square well Box potential is not realistic, thus consider a more realistic potential, the finite square well. At 0 < x < L, the particle is bound. According to classical mechanics, the particle in the well cannot penetrate the barrier potential U, however according to quantum mechanics, there is a probability for particles penetrates the potential. At x < 0 or x > L, the Schrödinger equation is : Since E < U, thus At x < 0: At x > L:
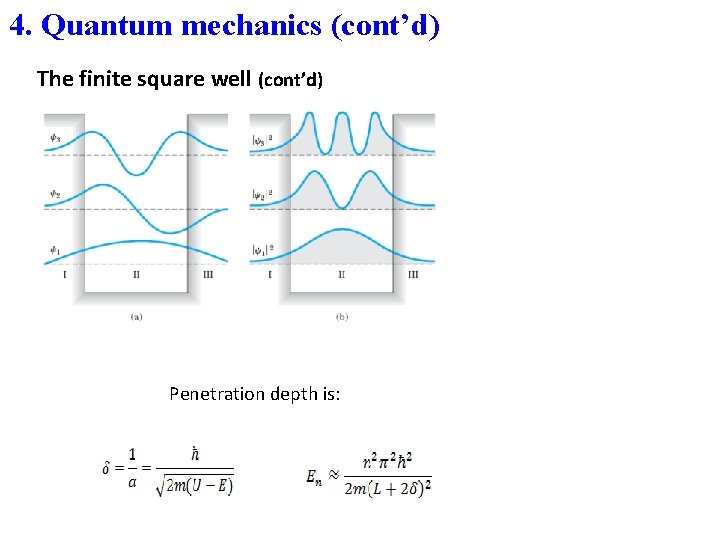
4. Quantum mechanics (cont’d) The finite square well (cont’d) Penetration depth is:
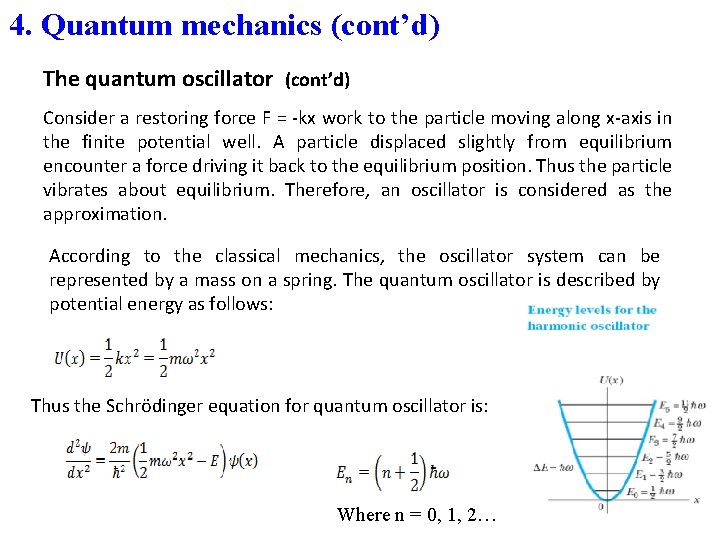
4. Quantum mechanics (cont’d) The quantum oscillator (cont’d) Consider a restoring force F = -kx work to the particle moving along x-axis in the finite potential well. A particle displaced slightly from equilibrium encounter a force driving it back to the equilibrium position. Thus the particle vibrates about equilibrium. Therefore, an oscillator is considered as the approximation. According to the classical mechanics, the oscillator system can be represented by a mass on a spring. The quantum oscillator is described by potential energy as follows: Thus the Schrödinger equation for quantum oscillator is: Where n = 0, 1, 2…
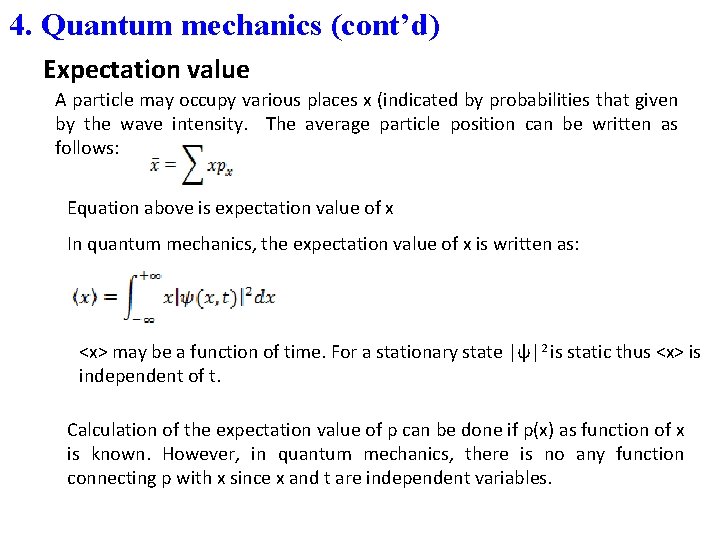
4. Quantum mechanics (cont’d) Expectation value A particle may occupy various places x (indicated by probabilities that given by the wave intensity. The average particle position can be written as follows: Equation above is expectation value of x In quantum mechanics, the expectation value of x is written as: <x> may be a function of time. For a stationary state |ψ|2 is static thus <x> is independent of t. Calculation of the expectation value of p can be done if p(x) as function of x is known. However, in quantum mechanics, there is no any function connecting p with x since x and t are independent variables.
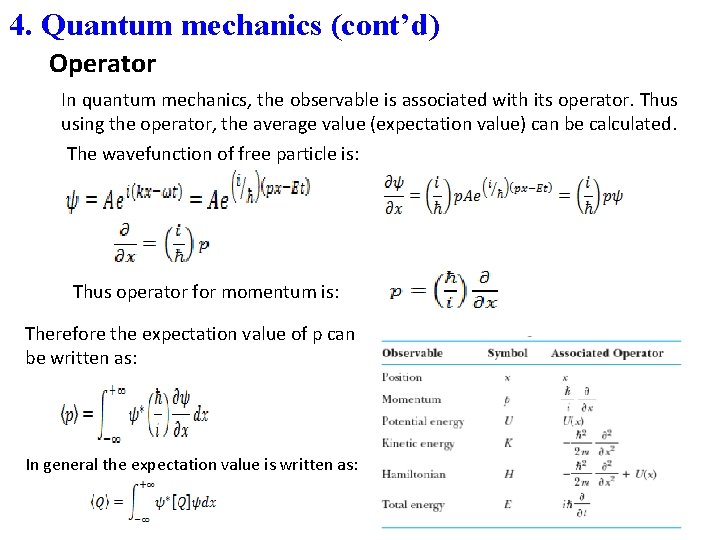
4. Quantum mechanics (cont’d) Operator In quantum mechanics, the observable is associated with its operator. Thus using the operator, the average value (expectation value) can be calculated. The wavefunction of free particle is: Thus operator for momentum is: Therefore the expectation value of p can be written as: In general the expectation value is written as:
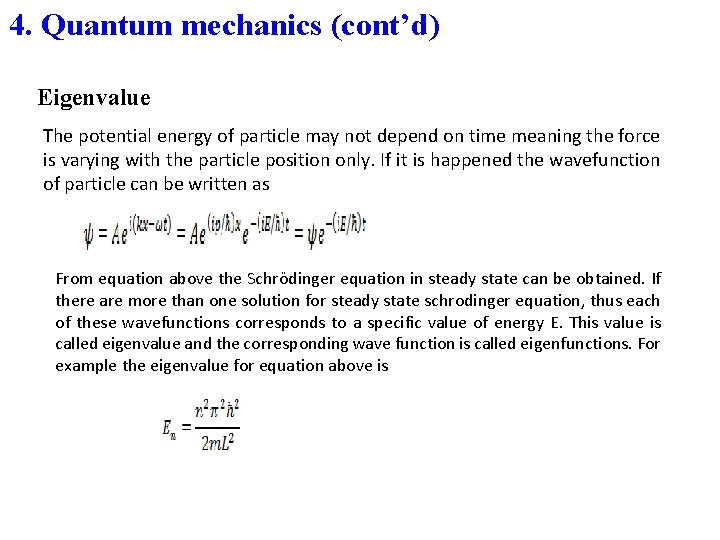
4. Quantum mechanics (cont’d) Eigenvalue The potential energy of particle may not depend on time meaning the force is varying with the particle position only. If it is happened the wavefunction of particle can be written as From equation above the Schrödinger equation in steady state can be obtained. If there are more than one solution for steady state schrodinger equation, thus each of these wavefunctions corresponds to a specific value of energy E. This value is called eigenvalue and the corresponding wave function is called eigenfunctions. For example the eigenvalue for equation above is
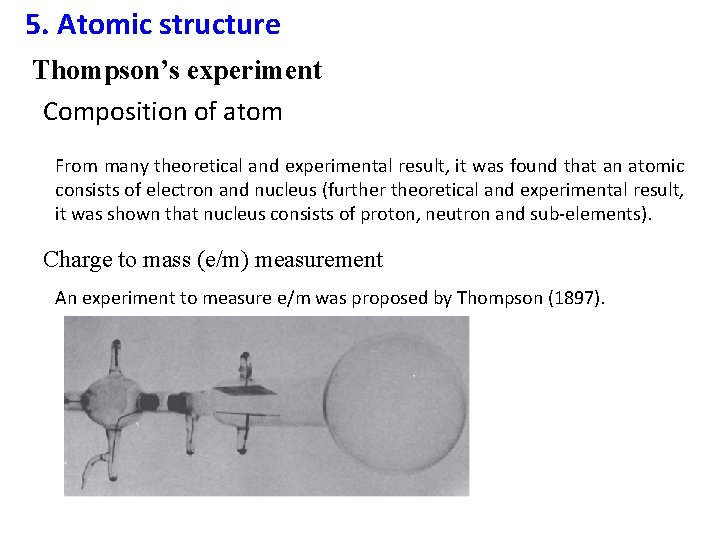
5. Atomic structure Thompson’s experiment Composition of atom From many theoretical and experimental result, it was found that an atomic consists of electron and nucleus (further theoretical and experimental result, it was shown that nucleus consists of proton, neutron and sub-elements). Charge to mass (e/m) measurement An experiment to measure e/m was proposed by Thompson (1897).
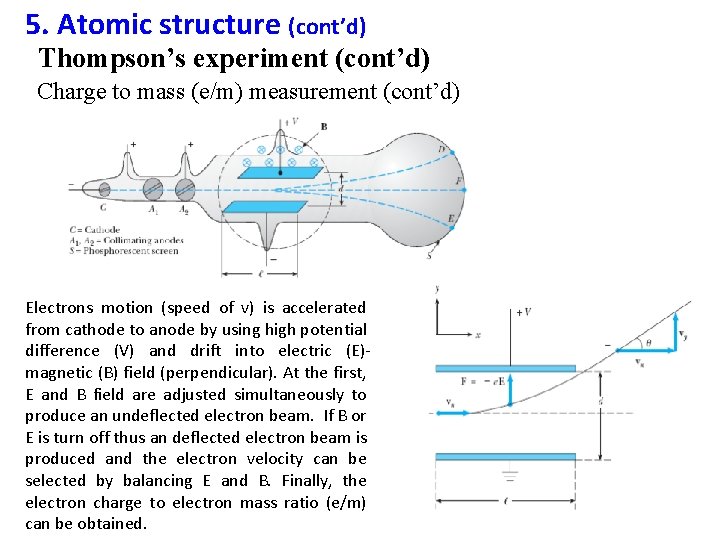
5. Atomic structure (cont’d) Thompson’s experiment (cont’d) Charge to mass (e/m) measurement (cont’d) Electrons motion (speed of v) is accelerated from cathode to anode by using high potential difference (V) and drift into electric (E)magnetic (B) field (perpendicular). At the first, E and B field are adjusted simultaneously to produce an undeflected electron beam. If B or E is turn off thus an deflected electron beam is produced and the electron velocity can be selected by balancing E and B. Finally, the electron charge to electron mass ratio (e/m) can be obtained.
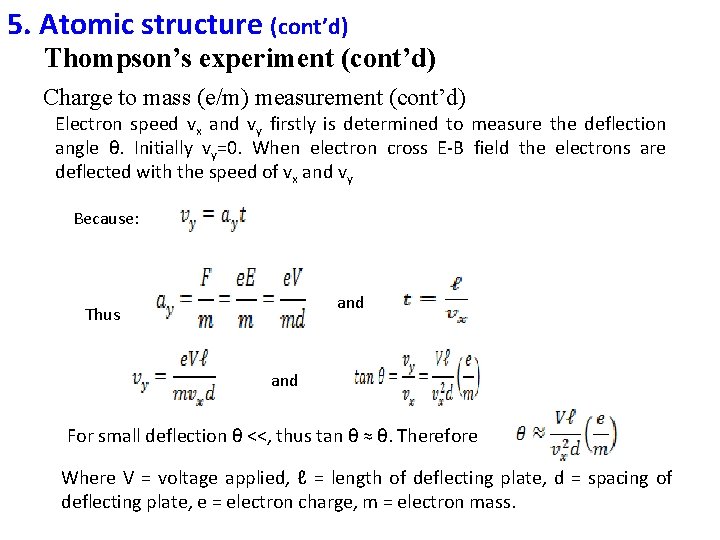
5. Atomic structure (cont’d) Thompson’s experiment (cont’d) Charge to mass (e/m) measurement (cont’d) Electron speed vx and vy firstly is determined to measure the deflection angle θ. Initially vy=0. When electron cross E-B field the electrons are deflected with the speed of vx and vy Because: and Thus and For small deflection θ <<, thus tan θ ≈ θ. Therefore Where V = voltage applied, ℓ = length of deflecting plate, d = spacing of deflecting plate, e = electron charge, m = electron mass.
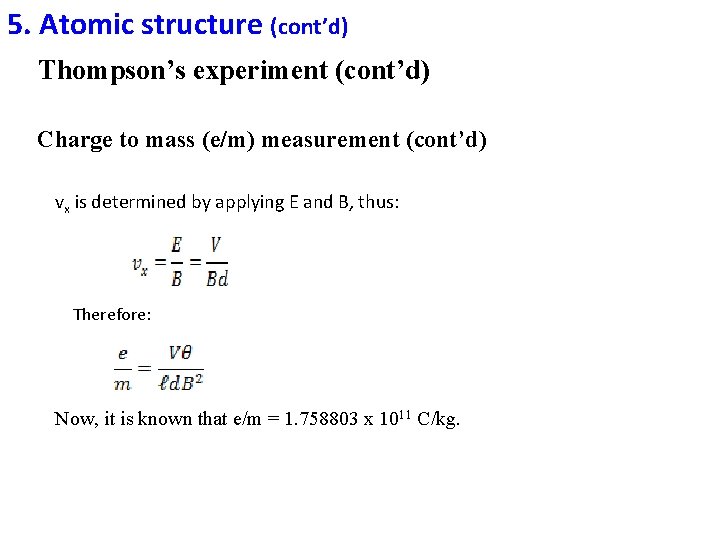
5. Atomic structure (cont’d) Thompson’s experiment (cont’d) Charge to mass (e/m) measurement (cont’d) vx is determined by applying E and B, thus: Therefore: Now, it is known that e/m = 1. 758803 x 1011 C/kg.
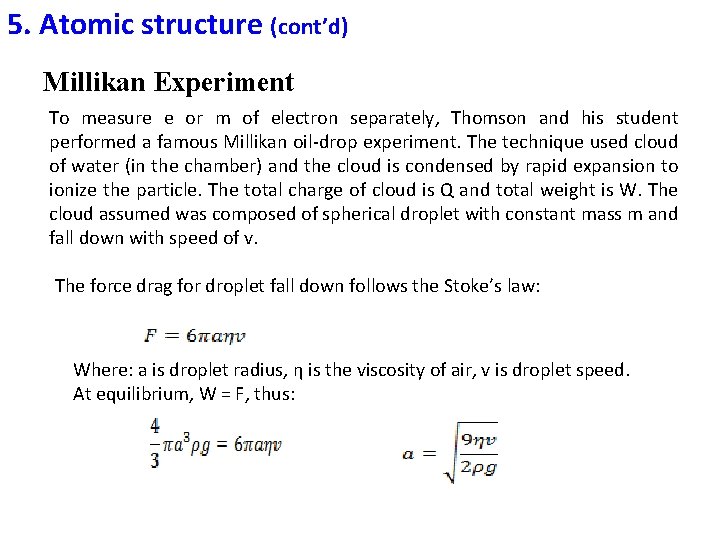
5. Atomic structure (cont’d) Millikan Experiment To measure e or m of electron separately, Thomson and his student performed a famous Millikan oil-drop experiment. The technique used cloud of water (in the chamber) and the cloud is condensed by rapid expansion to ionize the particle. The total charge of cloud is Q and total weight is W. The cloud assumed was composed of spherical droplet with constant mass m and fall down with speed of v. The force drag for droplet fall down follows the Stoke’s law: Where: a is droplet radius, η is the viscosity of air, v is droplet speed. At equilibrium, W = F, thus:
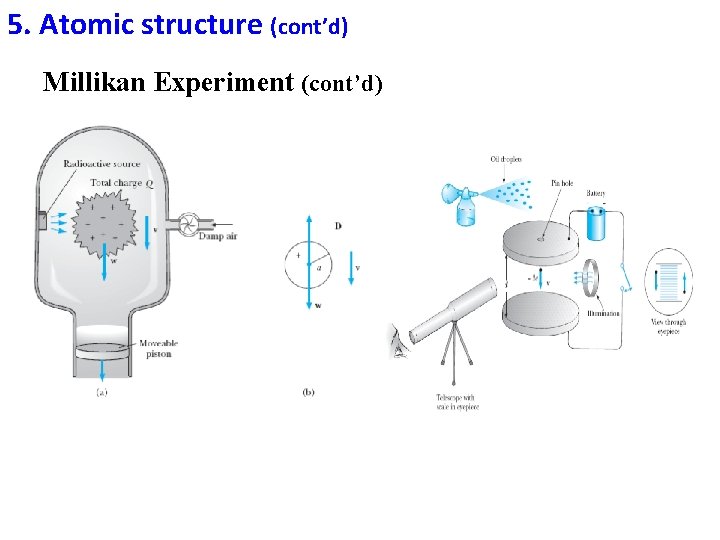
5. Atomic structure (cont’d) Millikan Experiment (cont’d)
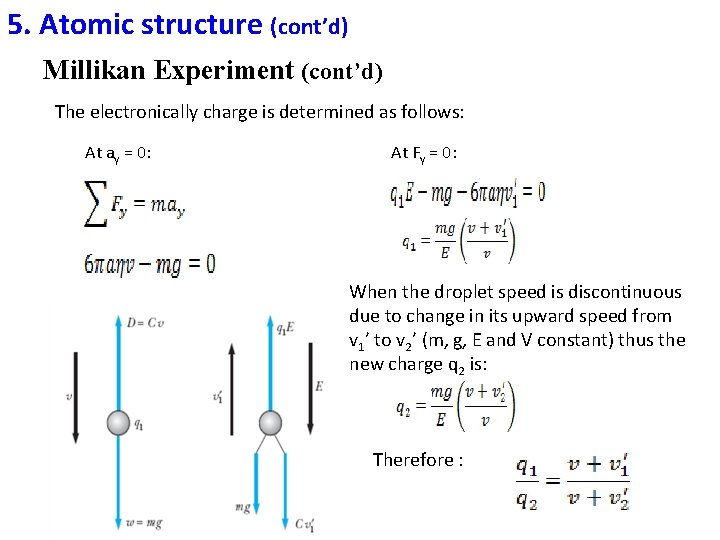
5. Atomic structure (cont’d) Millikan Experiment (cont’d) The electronically charge is determined as follows: At ay = 0: At Fy = 0: When the droplet speed is discontinuous due to change in its upward speed from v 1’ to v 2’ (m, g, E and V constant) thus the new charge q 2 is: Therefore :
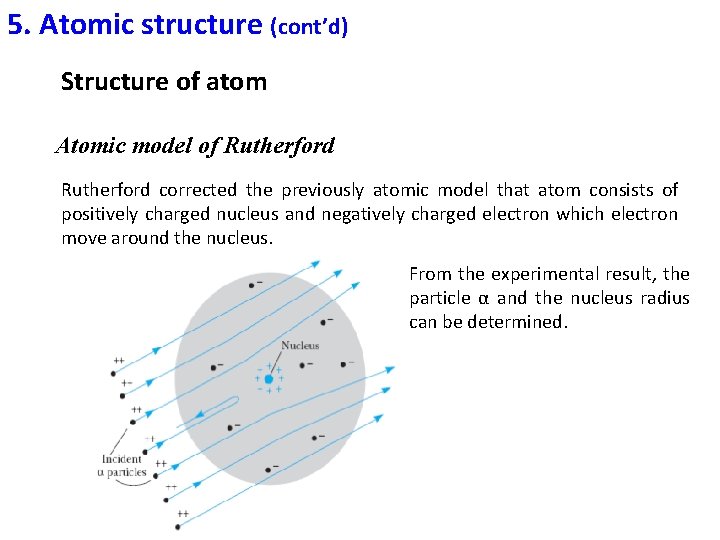
5. Atomic structure (cont’d) Structure of atom Atomic model of Rutherford corrected the previously atomic model that atom consists of positively charged nucleus and negatively charged electron which electron move around the nucleus. From the experimental result, the particle α and the nucleus radius can be determined.
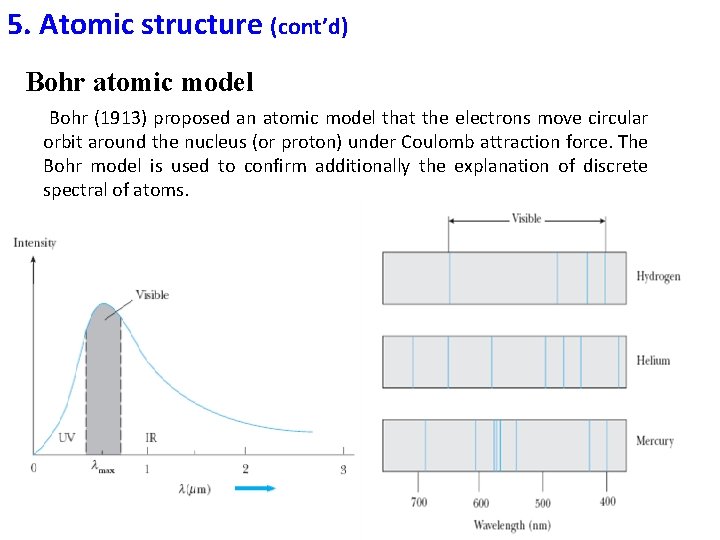
5. Atomic structure (cont’d) Bohr atomic model Bohr (1913) proposed an atomic model that the electrons move circular orbit around the nucleus (or proton) under Coulomb attraction force. The Bohr model is used to confirm additionally the explanation of discrete spectral of atoms.
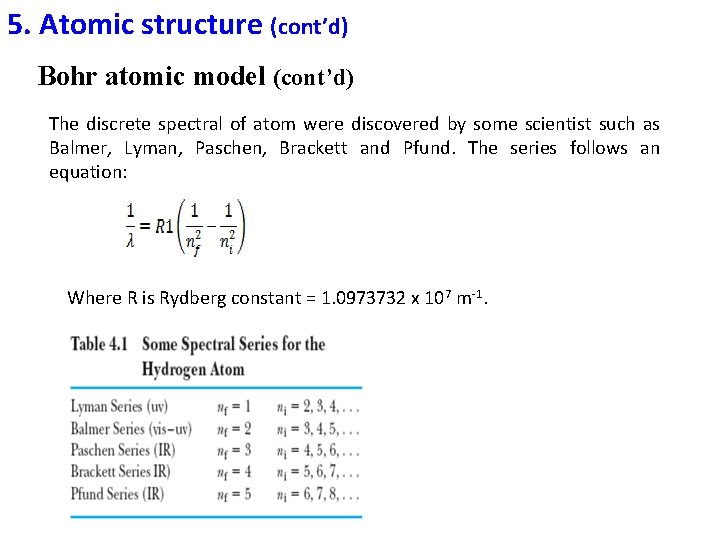
5. Atomic structure (cont’d) Bohr atomic model (cont’d) The discrete spectral of atom were discovered by some scientist such as Balmer, Lyman, Paschen, Brackett and Pfund. The series follows an equation: Where R is Rydberg constant = 1. 0973732 x 107 m-1.
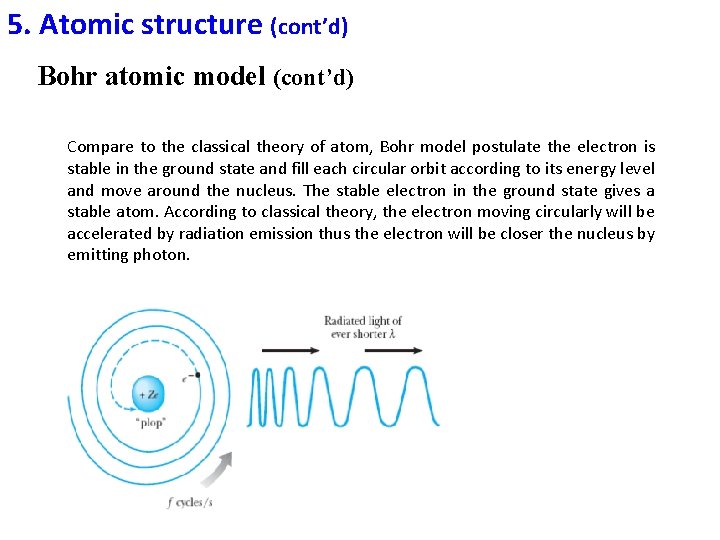
5. Atomic structure (cont’d) Bohr atomic model (cont’d) Compare to the classical theory of atom, Bohr model postulate the electron is stable in the ground state and fill each circular orbit according to its energy level and move around the nucleus. The stable electron in the ground state gives a stable atom. According to classical theory, the electron moving circularly will be accelerated by radiation emission thus the electron will be closer the nucleus by emitting photon.
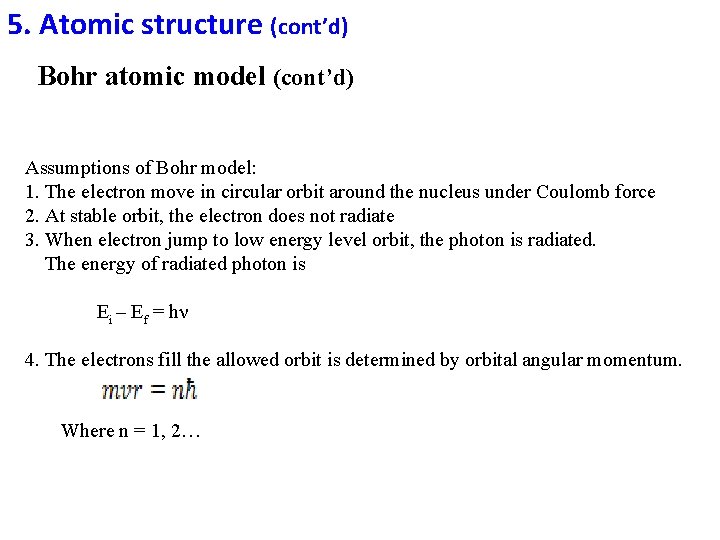
5. Atomic structure (cont’d) Bohr atomic model (cont’d) Assumptions of Bohr model: 1. The electron move in circular orbit around the nucleus under Coulomb force 2. At stable orbit, the electron does not radiate 3. When electron jump to low energy level orbit, the photon is radiated. The energy of radiated photon is Ei – Ef = hν 4. The electrons fill the allowed orbit is determined by orbital angular momentum. Where n = 1, 2…
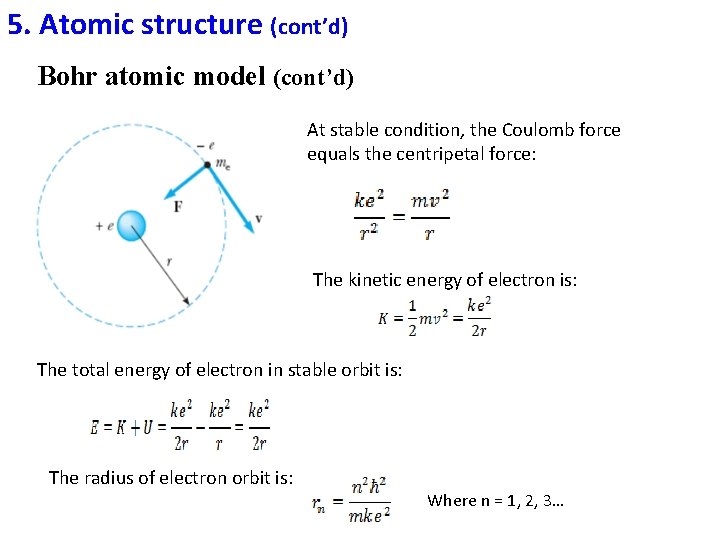
5. Atomic structure (cont’d) Bohr atomic model (cont’d) At stable condition, the Coulomb force equals the centripetal force: The kinetic energy of electron is: The total energy of electron in stable orbit is: The radius of electron orbit is: Where n = 1, 2, 3…
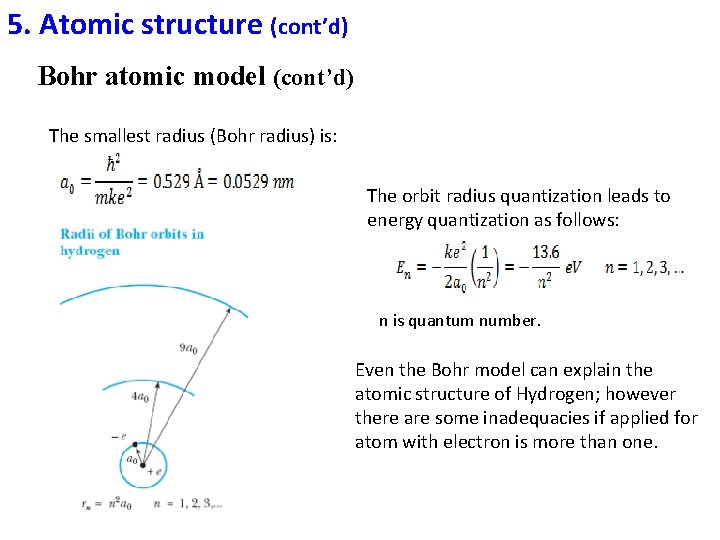
5. Atomic structure (cont’d) Bohr atomic model (cont’d) The smallest radius (Bohr radius) is: The orbit radius quantization leads to energy quantization as follows: n is quantum number. Even the Bohr model can explain the atomic structure of Hydrogen; however there are some inadequacies if applied for atom with electron is more than one.
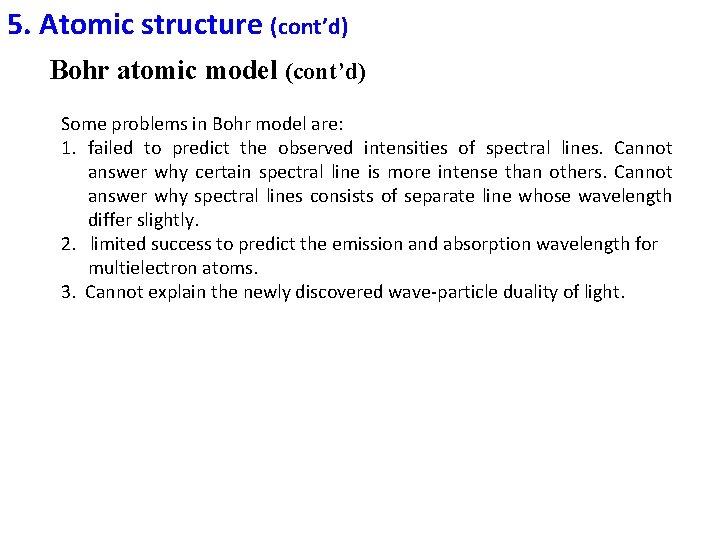
5. Atomic structure (cont’d) Bohr atomic model (cont’d) Some problems in Bohr model are: 1. failed to predict the observed intensities of spectral lines. Cannot answer why certain spectral line is more intense than others. Cannot answer why spectral lines consists of separate line whose wavelength differ slightly. 2. limited success to predict the emission and absorption wavelength for multielectron atoms. 3. Cannot explain the newly discovered wave-particle duality of light.
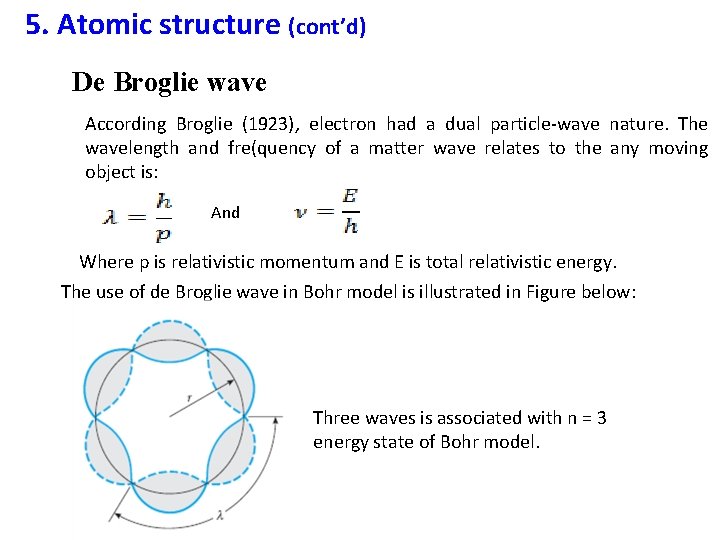
5. Atomic structure (cont’d) De Broglie wave According Broglie (1923), electron had a dual particle-wave nature. The wavelength and fre(quency of a matter wave relates to the any moving object is: And Where p is relativistic momentum and E is total relativistic energy. The use of de Broglie wave in Bohr model is illustrated in Figure below: Three waves is associated with n = 3 energy state of Bohr model.
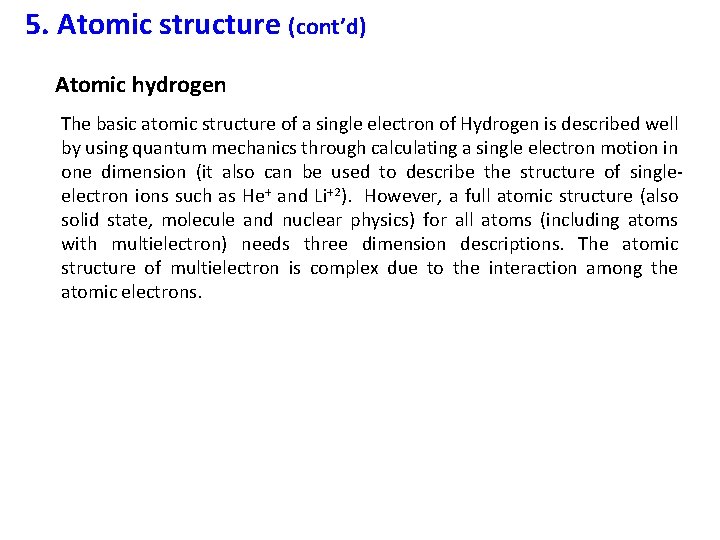
5. Atomic structure (cont’d) Atomic hydrogen The basic atomic structure of a single electron of Hydrogen is described well by using quantum mechanics through calculating a single electron motion in one dimension (it also can be used to describe the structure of singleelectron ions such as He+ and Li+2). However, a full atomic structure (also solid state, molecule and nuclear physics) for all atoms (including atoms with multielectron) needs three dimension descriptions. The atomic structure of multielectron is complex due to the interaction among the atomic electrons.
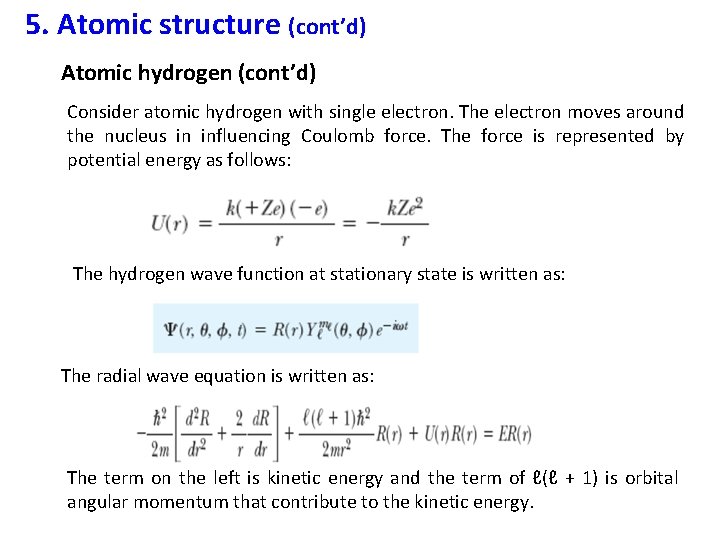
5. Atomic structure (cont’d) Atomic hydrogen (cont’d) Consider atomic hydrogen with single electron. The electron moves around the nucleus in influencing Coulomb force. The force is represented by potential energy as follows: The hydrogen wave function at stationary state is written as: The radial wave equation is written as: The term on the left is kinetic energy and the term of ℓ(ℓ + 1) is orbital angular momentum that contribute to the kinetic energy.
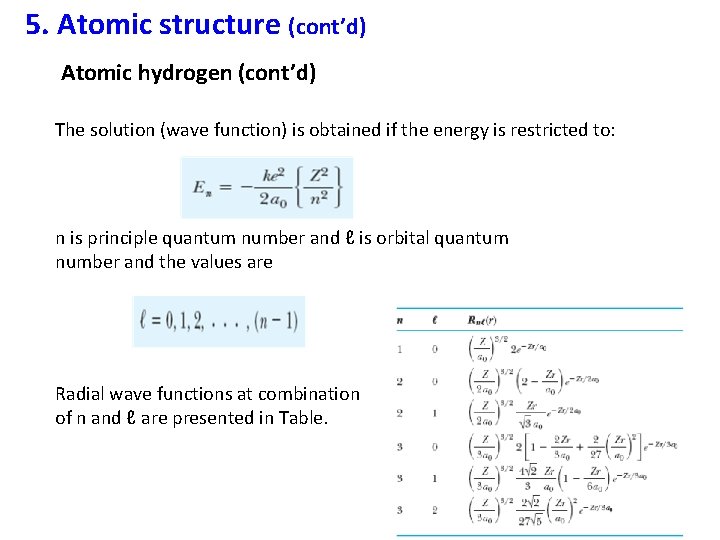
5. Atomic structure (cont’d) Atomic hydrogen (cont’d) The solution (wave function) is obtained if the energy is restricted to: n is principle quantum number and ℓ is orbital quantum number and the values are Radial wave functions at combination of n and ℓ are presented in Table.
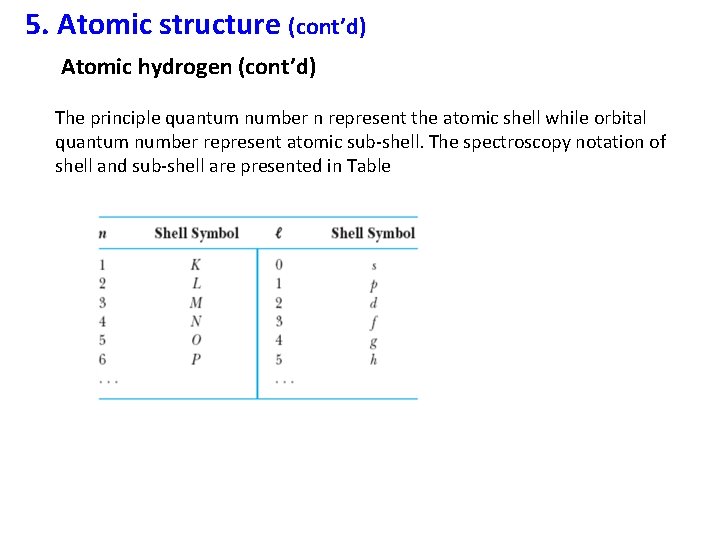
5. Atomic structure (cont’d) Atomic hydrogen (cont’d) The principle quantum number n represent the atomic shell while orbital quantum number represent atomic sub-shell. The spectroscopy notation of shell and sub-shell are presented in Table
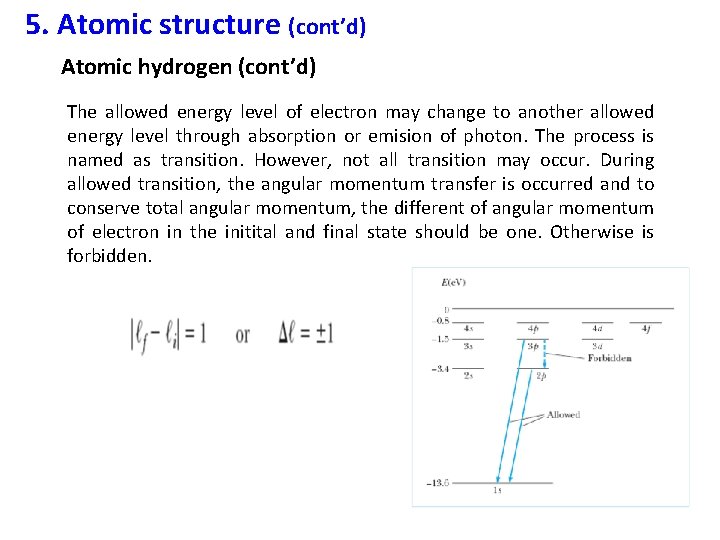
5. Atomic structure (cont’d) Atomic hydrogen (cont’d) The allowed energy level of electron may change to another allowed energy level through absorption or emision of photon. The process is named as transition. However, not all transition may occur. During allowed transition, the angular momentum transfer is occurred and to conserve total angular momentum, the different of angular momentum of electron in the initital and final state should be one. Otherwise is forbidden.
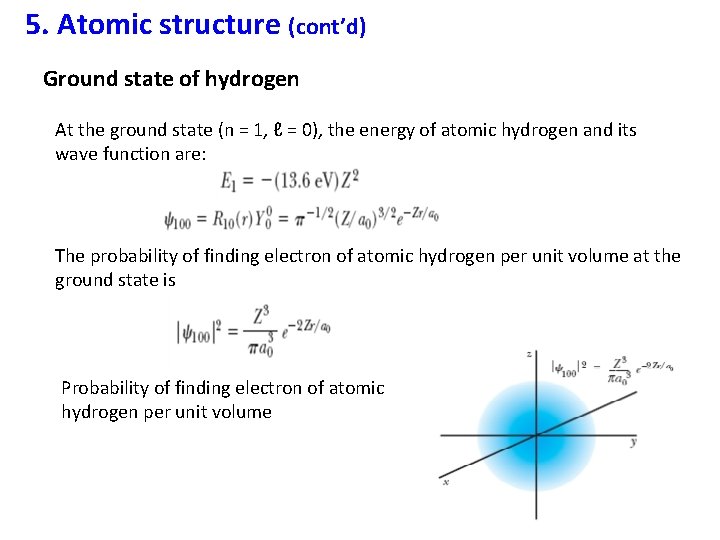
5. Atomic structure (cont’d) Ground state of hydrogen At the ground state (n = 1, ℓ = 0), the energy of atomic hydrogen and its wave function are: The probability of finding electron of atomic hydrogen per unit volume at the ground state is Probability of finding electron of atomic hydrogen per unit volume
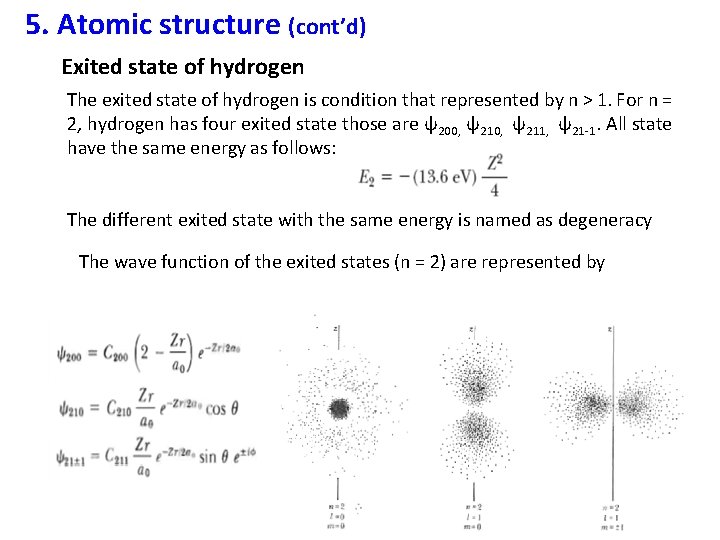
5. Atomic structure (cont’d) Exited state of hydrogen The exited state of hydrogen is condition that represented by n > 1. For n = 2, hydrogen has four exited state those are ψ200, ψ211, ψ21 -1. All state have the same energy as follows: The different exited state with the same energy is named as degeneracy The wave function of the exited states (n = 2) are represented by
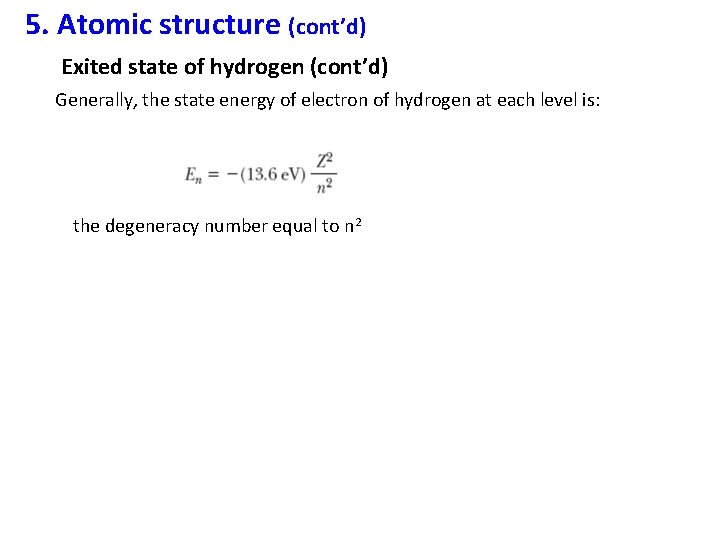
5. Atomic structure (cont’d) Exited state of hydrogen (cont’d) Generally, the state energy of electron of hydrogen at each level is: the degeneracy number equal to n 2
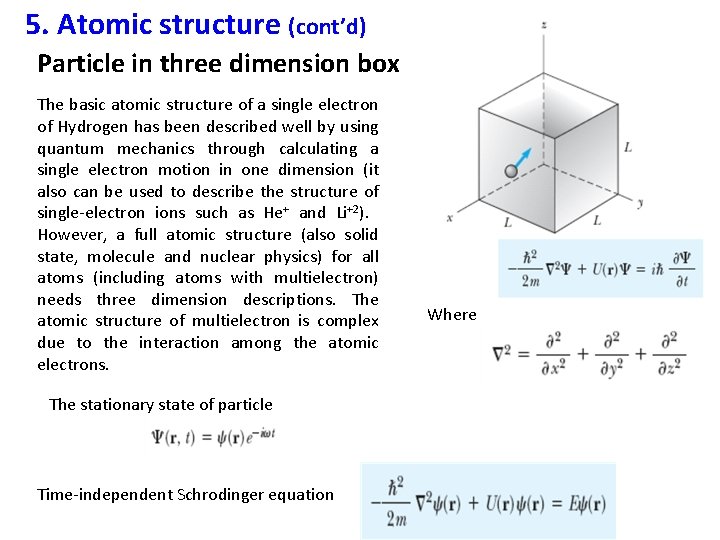
5. Atomic structure (cont’d) Particle in three dimension box The basic atomic structure of a single electron of Hydrogen has been described well by using quantum mechanics through calculating a single electron motion in one dimension (it also can be used to describe the structure of single-electron ions such as He+ and Li+2). However, a full atomic structure (also solid state, molecule and nuclear physics) for all atoms (including atoms with multielectron) needs three dimension descriptions. The atomic structure of multielectron is complex due to the interaction among the atomic electrons. The stationary state of particle Time-independent Schrodinger equation Where
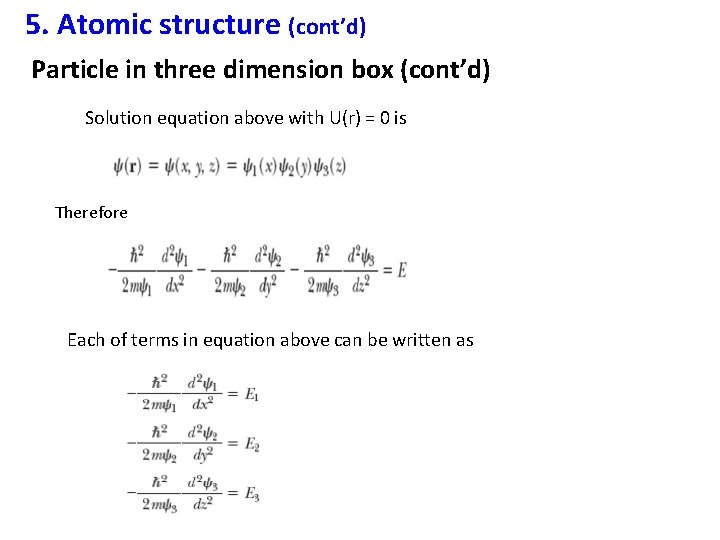
5. Atomic structure (cont’d) Particle in three dimension box (cont’d) Solution equation above with U(r) = 0 is Therefore Each of terms in equation above can be written as
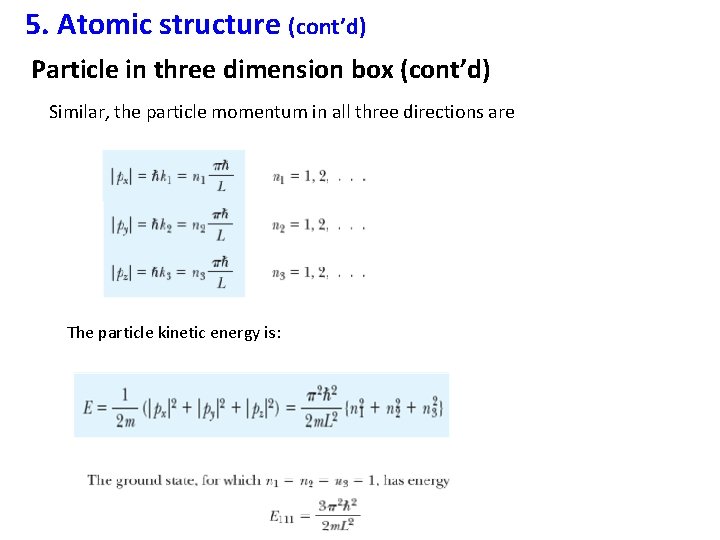
5. Atomic structure (cont’d) Particle in three dimension box (cont’d) Similar, the particle momentum in all three directions are The particle kinetic energy is:
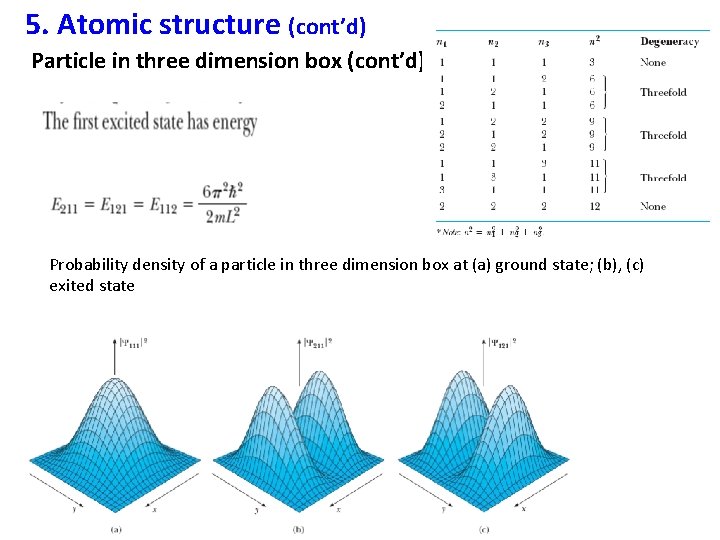
5. Atomic structure (cont’d) Particle in three dimension box (cont’d) Probability density of a particle in three dimension box at (a) ground state; (b), (c) exited state
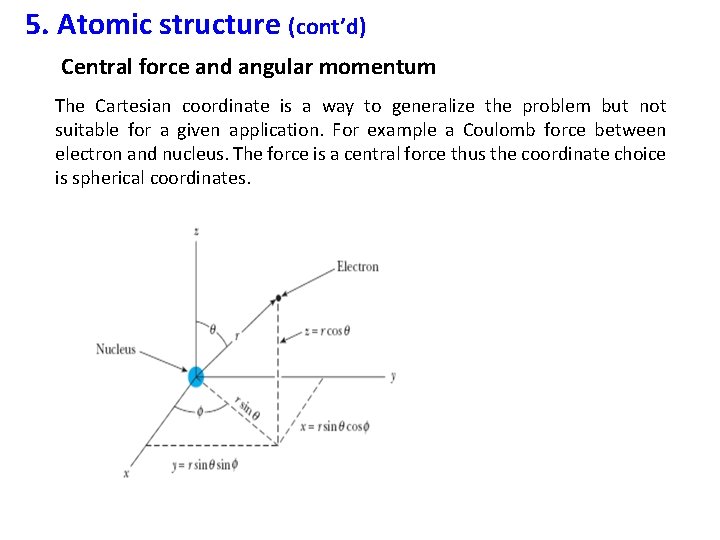
5. Atomic structure (cont’d) Central force and angular momentum The Cartesian coordinate is a way to generalize the problem but not suitable for a given application. For example a Coulomb force between electron and nucleus. The force is a central force thus the coordinate choice is spherical coordinates.
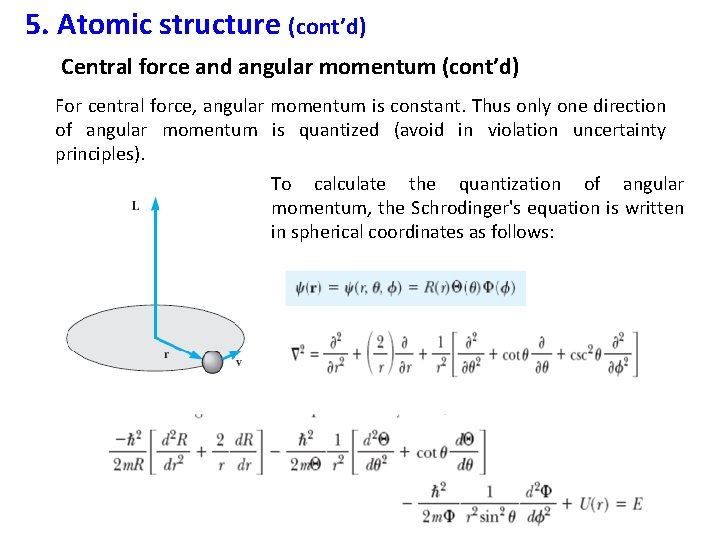
5. Atomic structure (cont’d) Central force and angular momentum (cont’d) For central force, angular momentum is constant. Thus only one direction of angular momentum is quantized (avoid in violation uncertainty principles). To calculate the quantization of angular momentum, the Schrodinger's equation is written in spherical coordinates as follows:
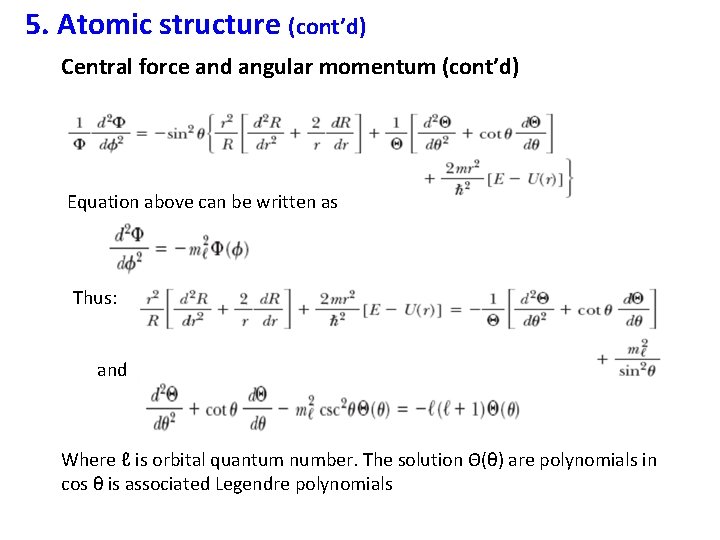
5. Atomic structure (cont’d) Central force and angular momentum (cont’d) Equation above can be written as Thus: and Where ℓ is orbital quantum number. The solution Θ(θ) are polynomials in cos θ is associated Legendre polynomials
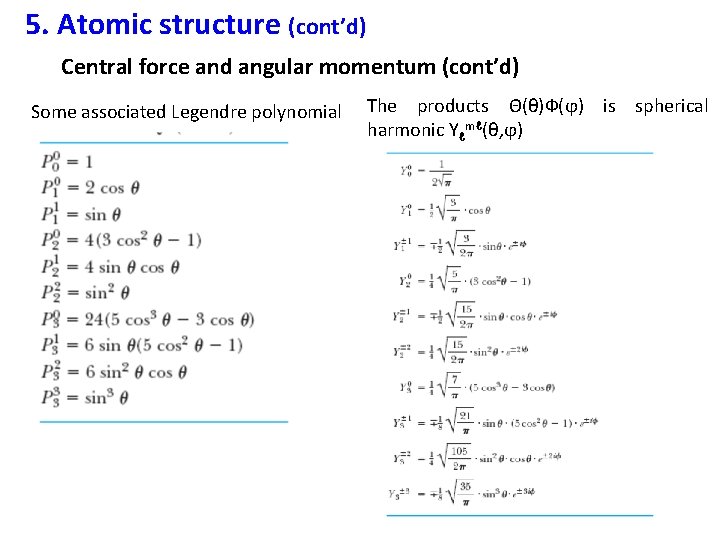
5. Atomic structure (cont’d) Central force and angular momentum (cont’d) Some associated Legendre polynomial The products Θ(θ)Ф(ϕ) is spherical harmonic Yℓmℓ(θ, ϕ)
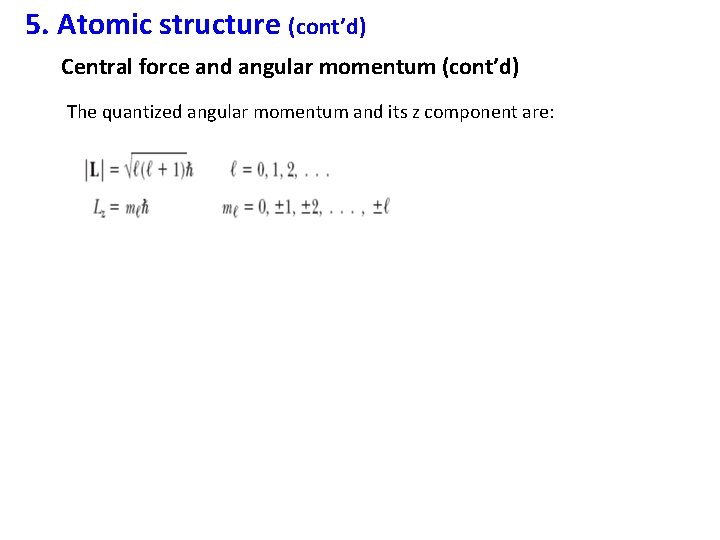
5. Atomic structure (cont’d) Central force and angular momentum (cont’d) The quantized angular momentum and its z component are:
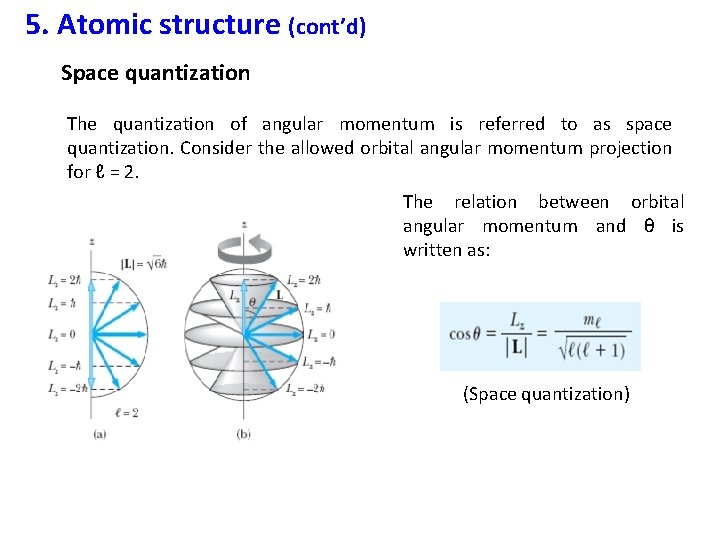
5. Atomic structure (cont’d) Space quantization The quantization of angular momentum is referred to as space quantization. Consider the allowed orbital angular momentum projection for ℓ = 2. The relation between orbital angular momentum and θ is written as: (Space quantization)
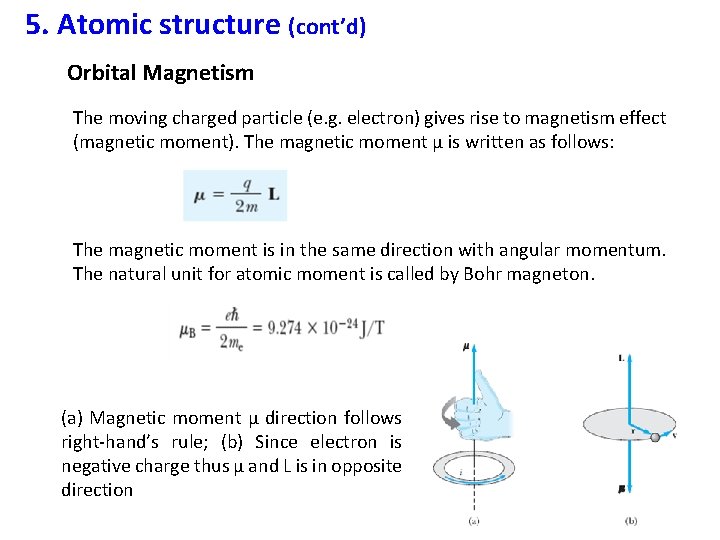
5. Atomic structure (cont’d) Orbital Magnetism The moving charged particle (e. g. electron) gives rise to magnetism effect (magnetic moment). The magnetic moment μ is written as follows: The magnetic moment is in the same direction with angular momentum. The natural unit for atomic moment is called by Bohr magneton. (a) Magnetic moment μ direction follows right-hand’s rule; (b) Since electron is negative charge thus μ and L is in opposite direction
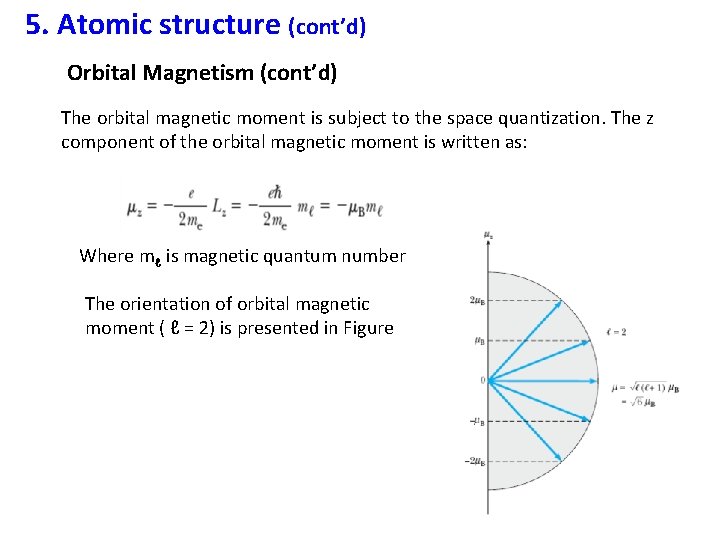
5. Atomic structure (cont’d) Orbital Magnetism (cont’d) The orbital magnetic moment is subject to the space quantization. The z component of the orbital magnetic moment is written as: Where mℓ is magnetic quantum number The orientation of orbital magnetic moment ( ℓ = 2) is presented in Figure
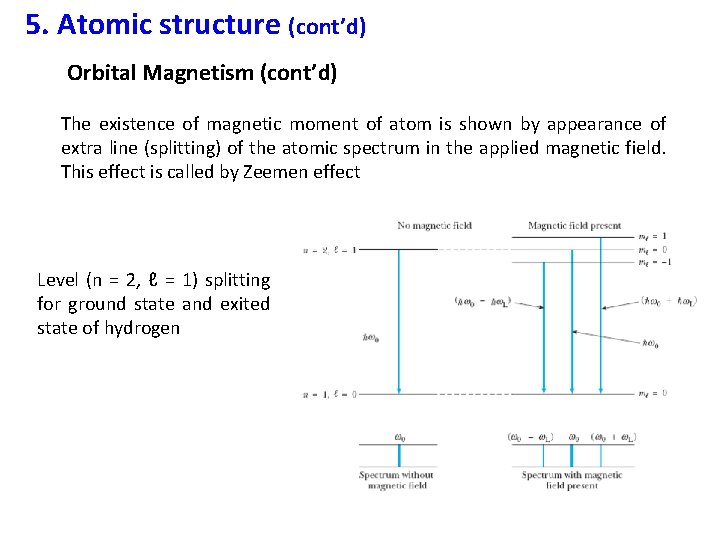
5. Atomic structure (cont’d) Orbital Magnetism (cont’d) The existence of magnetic moment of atom is shown by appearance of extra line (splitting) of the atomic spectrum in the applied magnetic field. This effect is called by Zeemen effect Level (n = 2, ℓ = 1) splitting for ground state and exited state of hydrogen
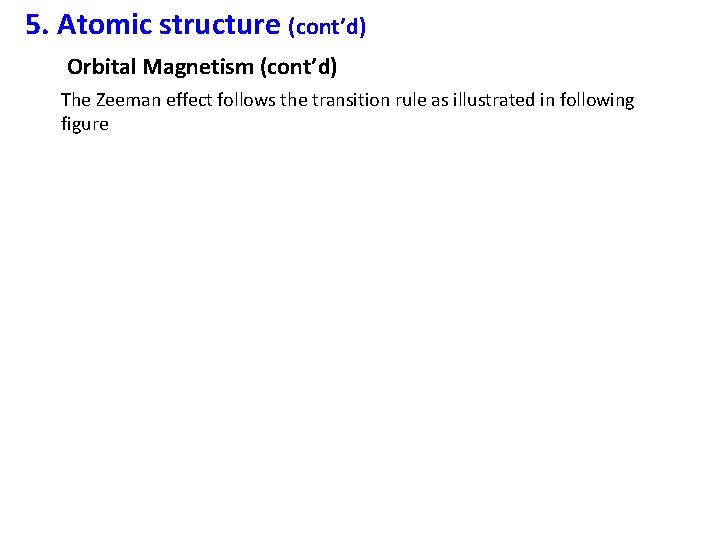
5. Atomic structure (cont’d) Orbital Magnetism (cont’d) The Zeeman effect follows the transition rule as illustrated in following figure
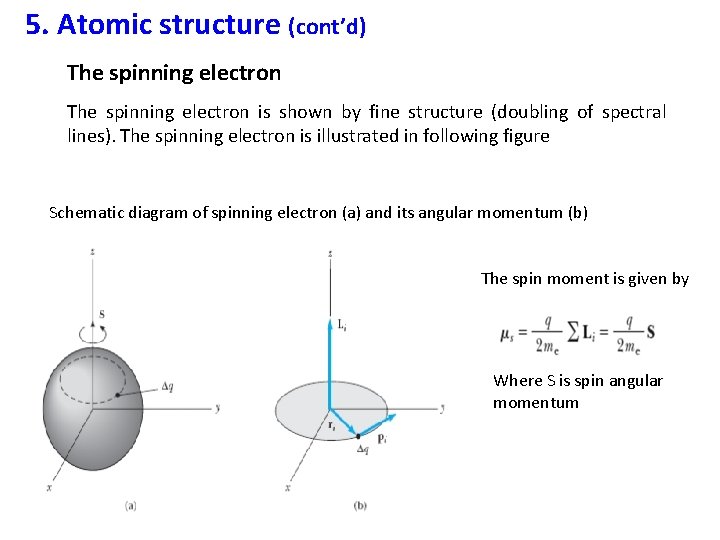
5. Atomic structure (cont’d) The spinning electron is shown by fine structure (doubling of spectral lines). The spinning electron is illustrated in following figure Schematic diagram of spinning electron (a) and its angular momentum (b) The spin moment is given by Where S is spin angular momentum
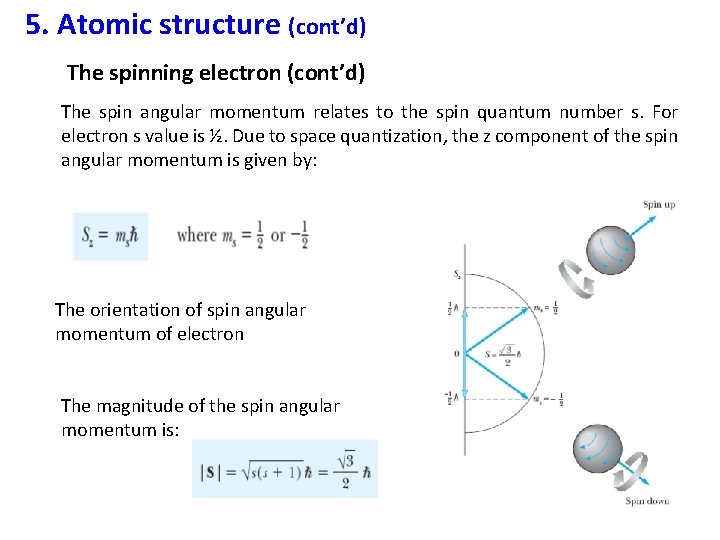
5. Atomic structure (cont’d) The spinning electron (cont’d) The spin angular momentum relates to the spin quantum number s. For electron s value is ½. Due to space quantization, the z component of the spin angular momentum is given by: The orientation of spin angular momentum of electron The magnitude of the spin angular momentum is:
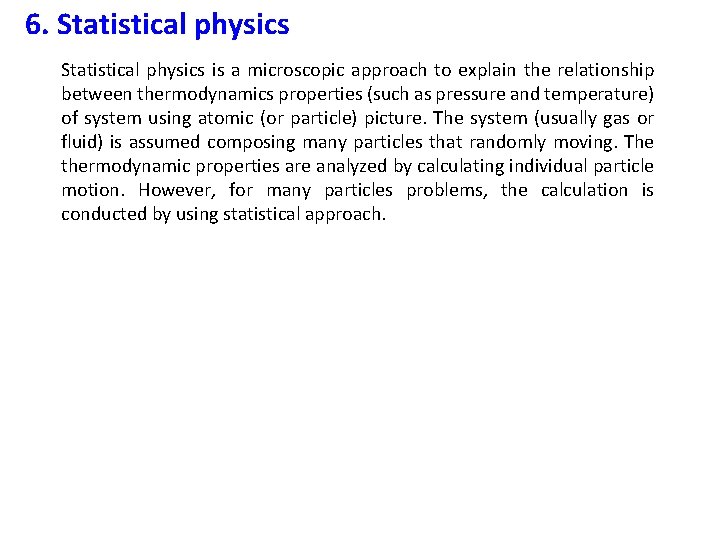
6. Statistical physics is a microscopic approach to explain the relationship between thermodynamics properties (such as pressure and temperature) of system using atomic (or particle) picture. The system (usually gas or fluid) is assumed composing many particles that randomly moving. The thermodynamic properties are analyzed by calculating individual particle motion. However, for many particles problems, the calculation is conducted by using statistical approach.
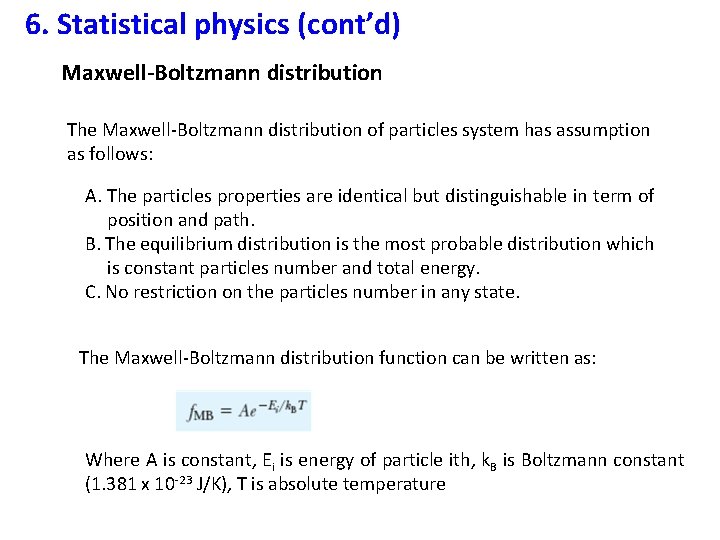
6. Statistical physics (cont’d) Maxwell-Boltzmann distribution The Maxwell-Boltzmann distribution of particles system has assumption as follows: A. The particles properties are identical but distinguishable in term of position and path. B. The equilibrium distribution is the most probable distribution which is constant particles number and total energy. C. No restriction on the particles number in any state. The Maxwell-Boltzmann distribution function can be written as: Where A is constant, Ei is energy of particle ith, k. B is Boltzmann constant (1. 381 x 10 -23 J/K), T is absolute temperature
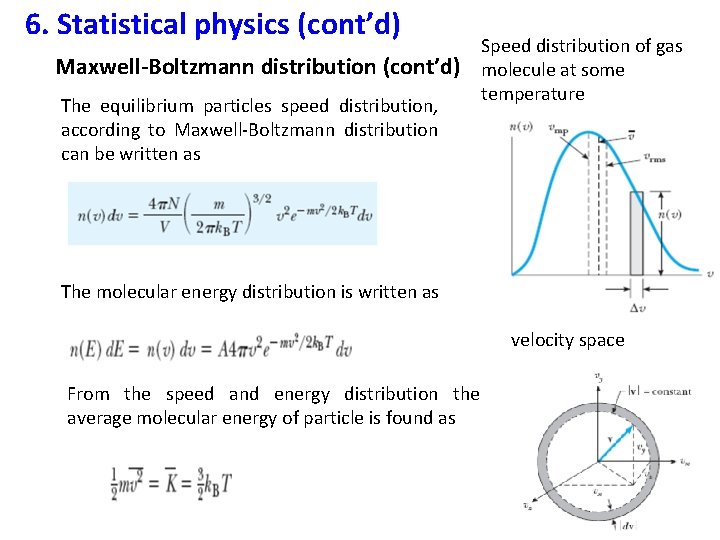
6. Statistical physics (cont’d) Maxwell-Boltzmann distribution (cont’d) The equilibrium particles speed distribution, according to Maxwell-Boltzmann distribution can be written as Speed distribution of gas molecule at some temperature The molecular energy distribution is written as velocity space From the speed and energy distribution the average molecular energy of particle is found as
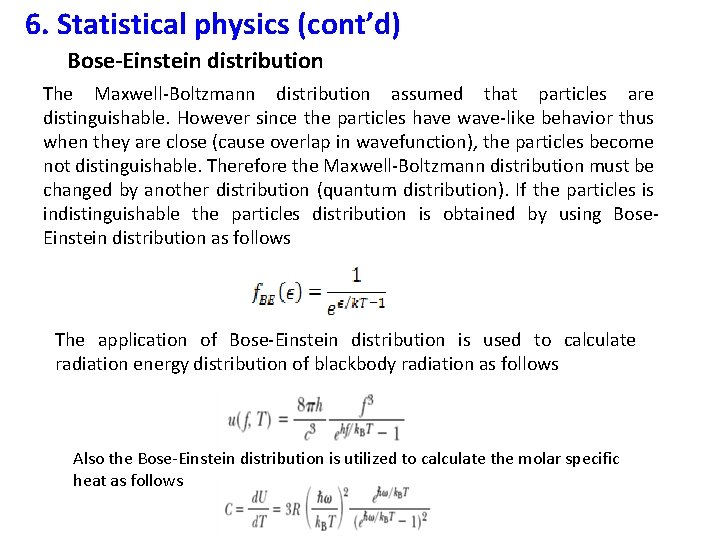
6. Statistical physics (cont’d) Bose-Einstein distribution The Maxwell-Boltzmann distribution assumed that particles are distinguishable. However since the particles have wave-like behavior thus when they are close (cause overlap in wavefunction), the particles become not distinguishable. Therefore the Maxwell-Boltzmann distribution must be changed by another distribution (quantum distribution). If the particles is indistinguishable the particles distribution is obtained by using Bose. Einstein distribution as follows The application of Bose-Einstein distribution is used to calculate radiation energy distribution of blackbody radiation as follows Also the Bose-Einstein distribution is utilized to calculate the molar specific heat as follows
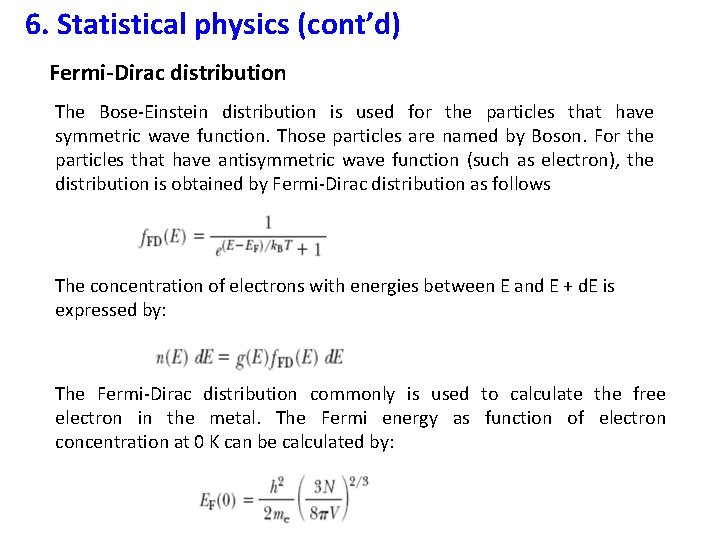
6. Statistical physics (cont’d) Fermi-Dirac distribution The Bose-Einstein distribution is used for the particles that have symmetric wave function. Those particles are named by Boson. For the particles that have antisymmetric wave function (such as electron), the distribution is obtained by Fermi-Dirac distribution as follows The concentration of electrons with energies between E and E + d. E is expressed by: The Fermi-Dirac distribution commonly is used to calculate the free electron in the metal. The Fermi energy as function of electron concentration at 0 K can be calculated by:
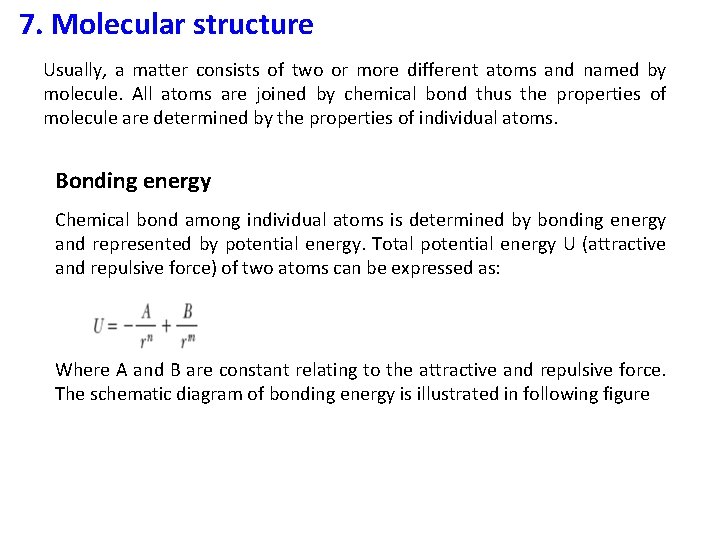
7. Molecular structure Usually, a matter consists of two or more different atoms and named by molecule. All atoms are joined by chemical bond thus the properties of molecule are determined by the properties of individual atoms. Bonding energy Chemical bond among individual atoms is determined by bonding energy and represented by potential energy. Total potential energy U (attractive and repulsive force) of two atoms can be expressed as: Where A and B are constant relating to the attractive and repulsive force. The schematic diagram of bonding energy is illustrated in following figure
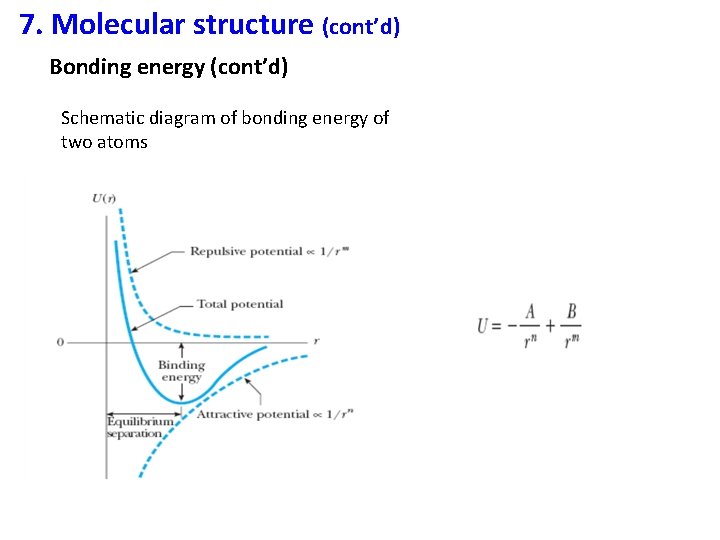
7. Molecular structure (cont’d) Bonding energy (cont’d) Schematic diagram of bonding energy of two atoms
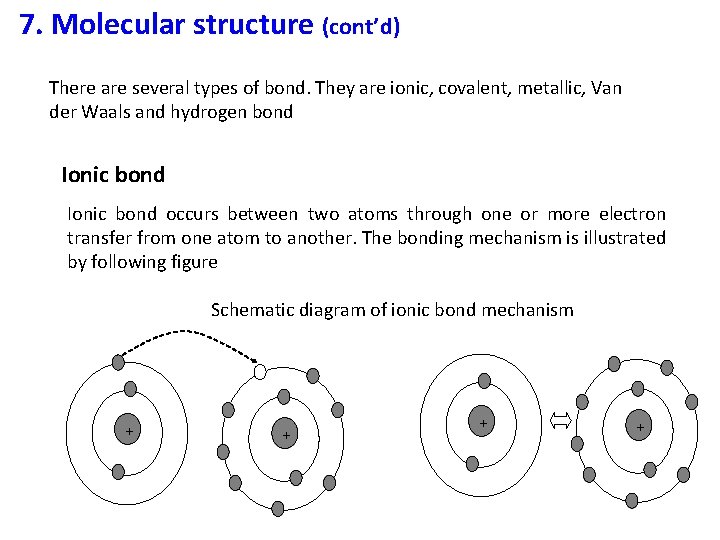
7. Molecular structure (cont’d) There are several types of bond. They are ionic, covalent, metallic, Van der Waals and hydrogen bond Ionic bond occurs between two atoms through one or more electron transfer from one atom to another. The bonding mechanism is illustrated by following figure Schematic diagram of ionic bond mechanism + +
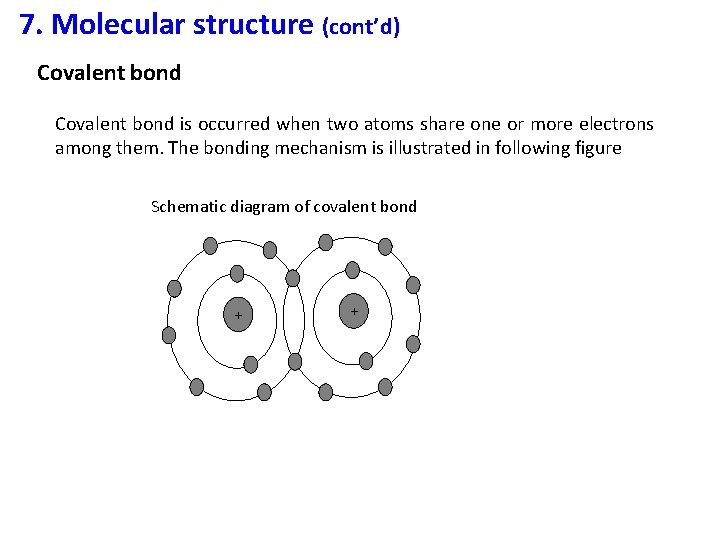
7. Molecular structure (cont’d) Covalent bond is occurred when two atoms share one or more electrons among them. The bonding mechanism is illustrated in following figure Schematic diagram of covalent bond + +
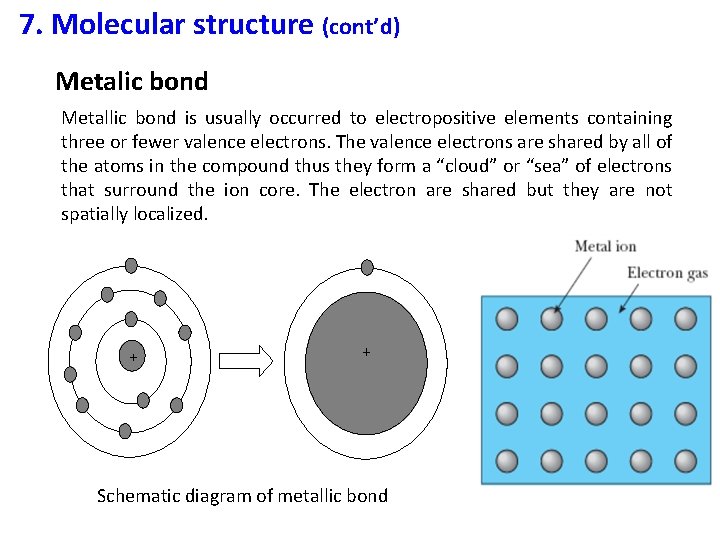
7. Molecular structure (cont’d) Metalic bond Metallic bond is usually occurred to electropositive elements containing three or fewer valence electrons. The valence electrons are shared by all of the atoms in the compound thus they form a “cloud” or “sea” of electrons that surround the ion core. The electron are shared but they are not spatially localized. + + Schematic diagram of metallic bond
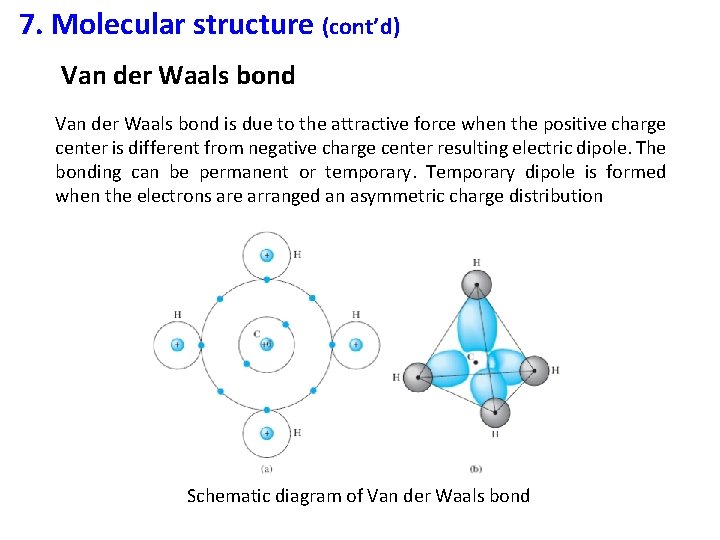
7. Molecular structure (cont’d) Van der Waals bond is due to the attractive force when the positive charge center is different from negative charge center resulting electric dipole. The bonding can be permanent or temporary. Temporary dipole is formed when the electrons are arranged an asymmetric charge distribution Schematic diagram of Van der Waals bond
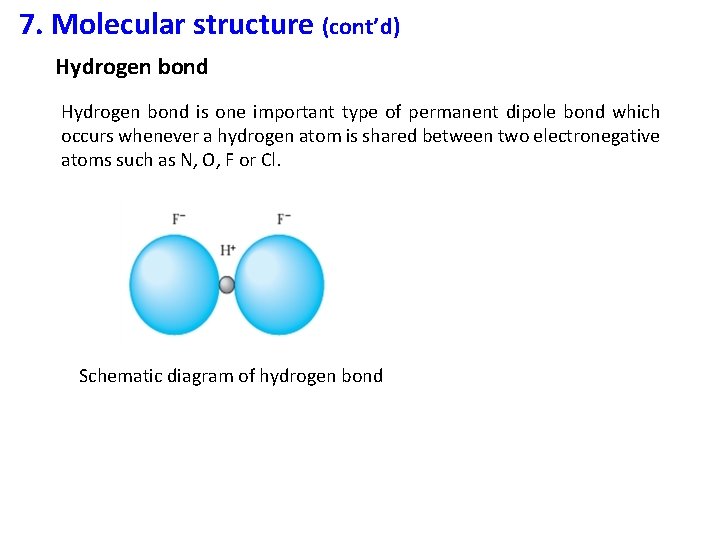
7. Molecular structure (cont’d) Hydrogen bond is one important type of permanent dipole bond which occurs whenever a hydrogen atom is shared between two electronegative atoms such as N, O, F or Cl. Schematic diagram of hydrogen bond
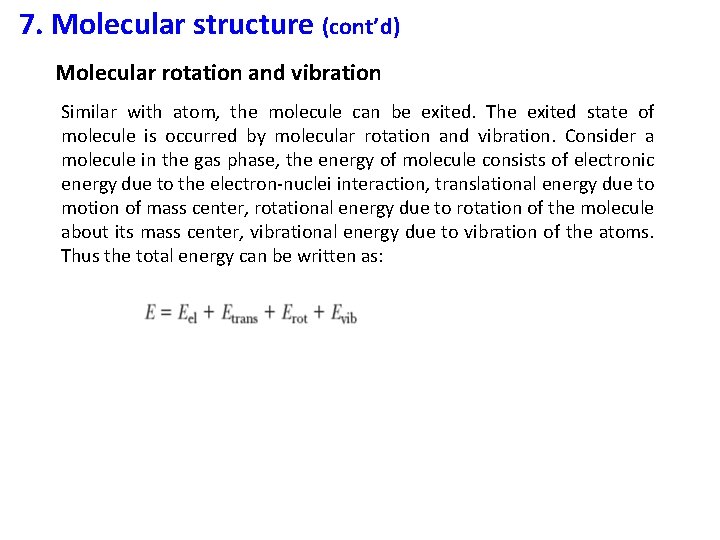
7. Molecular structure (cont’d) Molecular rotation and vibration Similar with atom, the molecule can be exited. The exited state of molecule is occurred by molecular rotation and vibration. Consider a molecule in the gas phase, the energy of molecule consists of electronic energy due to the electron-nuclei interaction, translational energy due to motion of mass center, rotational energy due to rotation of the molecule about its mass center, vibrational energy due to vibration of the atoms. Thus the total energy can be written as:
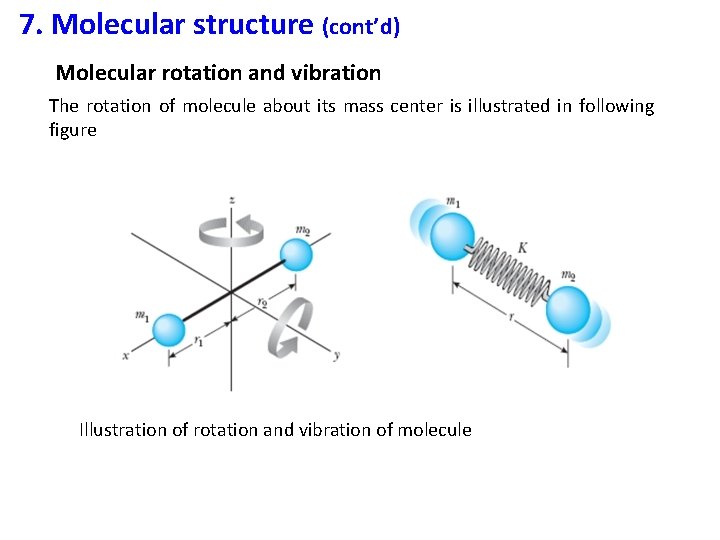
7. Molecular structure (cont’d) Molecular rotation and vibration The rotation of molecule about its mass center is illustrated in following figure Illustration of rotation and vibration of molecule
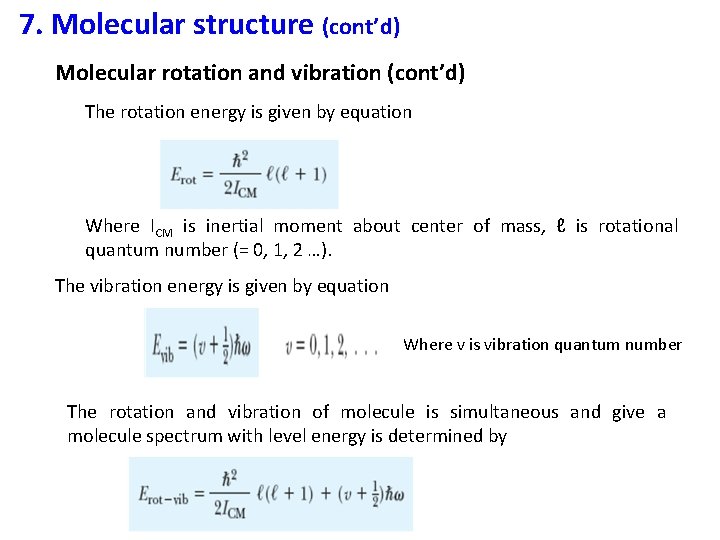
7. Molecular structure (cont’d) Molecular rotation and vibration (cont’d) The rotation energy is given by equation Where ICM is inertial moment about center of mass, ℓ is rotational quantum number (= 0, 1, 2 …). The vibration energy is given by equation Where v is vibration quantum number The rotation and vibration of molecule is simultaneous and give a molecule spectrum with level energy is determined by
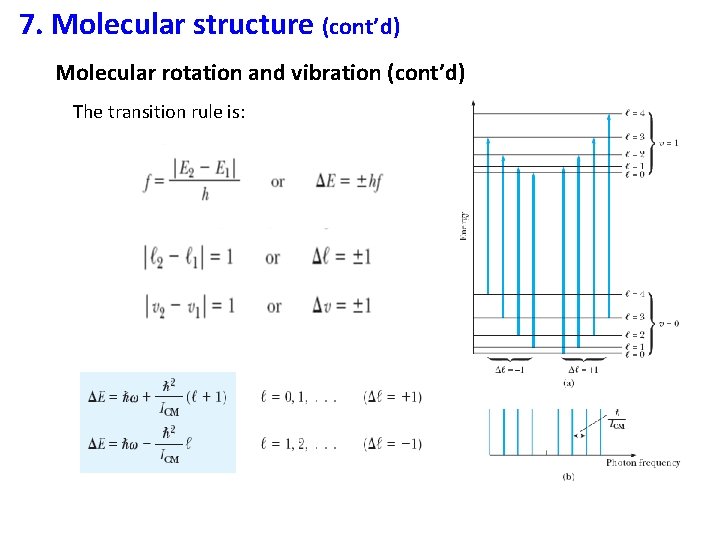
7. Molecular structure (cont’d) Molecular rotation and vibration (cont’d) The transition rule is:
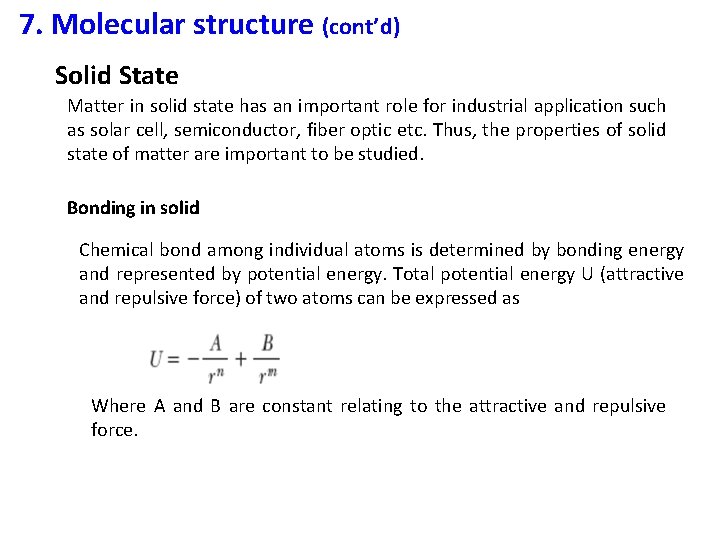
7. Molecular structure (cont’d) Solid State Matter in solid state has an important role for industrial application such as solar cell, semiconductor, fiber optic etc. Thus, the properties of solid state of matter are important to be studied. Bonding in solid Chemical bond among individual atoms is determined by bonding energy and represented by potential energy. Total potential energy U (attractive and repulsive force) of two atoms can be expressed as Where A and B are constant relating to the attractive and repulsive force.
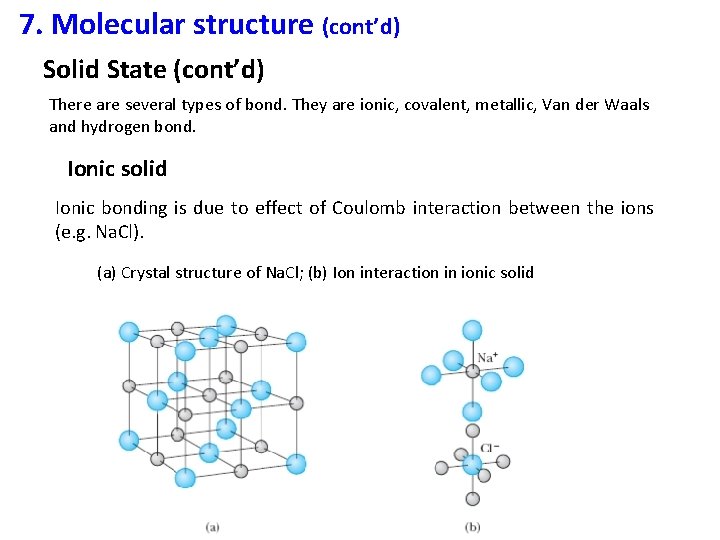
7. Molecular structure (cont’d) Solid State (cont’d) There are several types of bond. They are ionic, covalent, metallic, Van der Waals and hydrogen bond. Ionic solid Ionic bonding is due to effect of Coulomb interaction between the ions (e. g. Na. Cl). (a) Crystal structure of Na. Cl; (b) Ion interaction in ionic solid
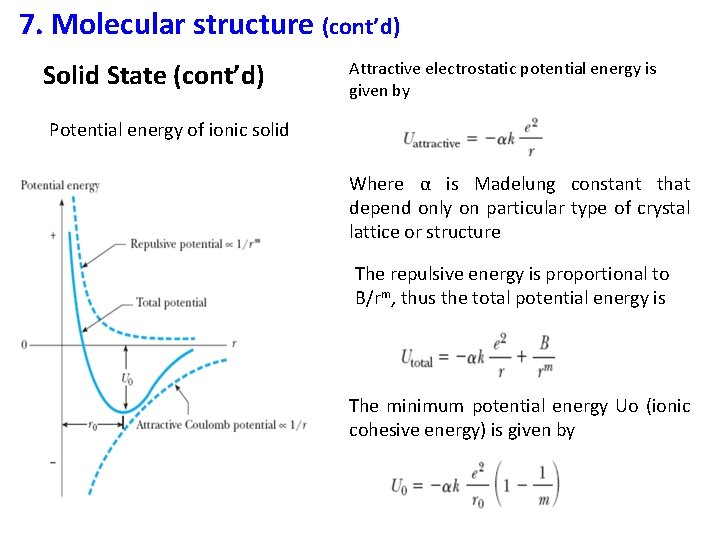
7. Molecular structure (cont’d) Solid State (cont’d) Attractive electrostatic potential energy is given by Potential energy of ionic solid Where α is Madelung constant that depend only on particular type of crystal lattice or structure The repulsive energy is proportional to B/rm, thus the total potential energy is The minimum potential energy Uo (ionic cohesive energy) is given by
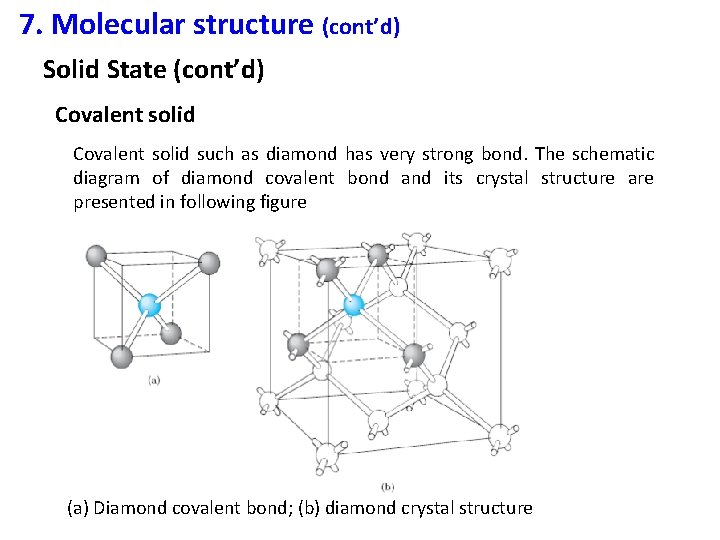
7. Molecular structure (cont’d) Solid State (cont’d) Covalent solid such as diamond has very strong bond. The schematic diagram of diamond covalent bond and its crystal structure are presented in following figure (a) Diamond covalent bond; (b) diamond crystal structure
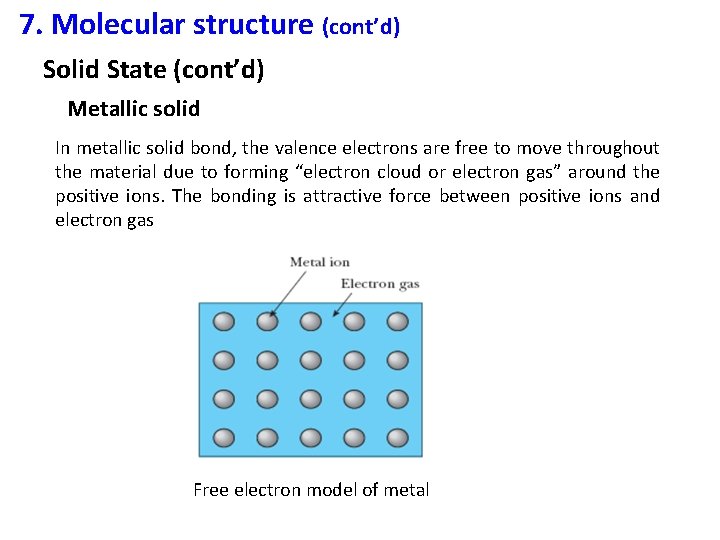
7. Molecular structure (cont’d) Solid State (cont’d) Metallic solid In metallic solid bond, the valence electrons are free to move throughout the material due to forming “electron cloud or electron gas” around the positive ions. The bonding is attractive force between positive ions and electron gas Free electron model of metal
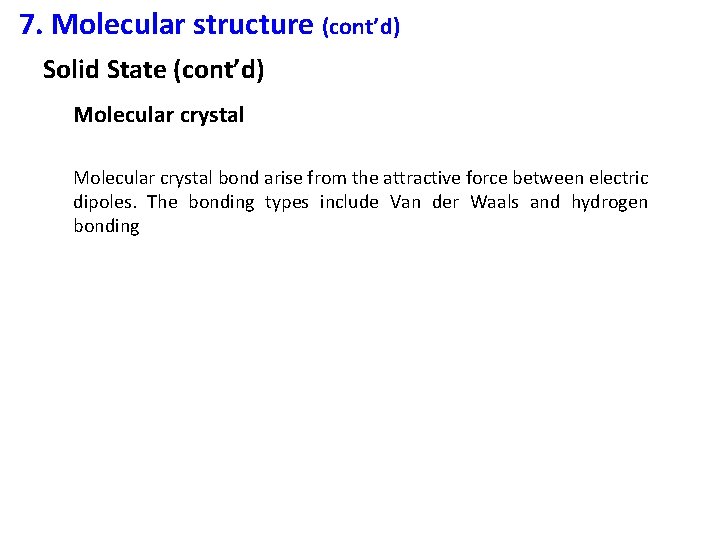
7. Molecular structure (cont’d) Solid State (cont’d) Molecular crystal bond arise from the attractive force between electric dipoles. The bonding types include Van der Waals and hydrogen bonding
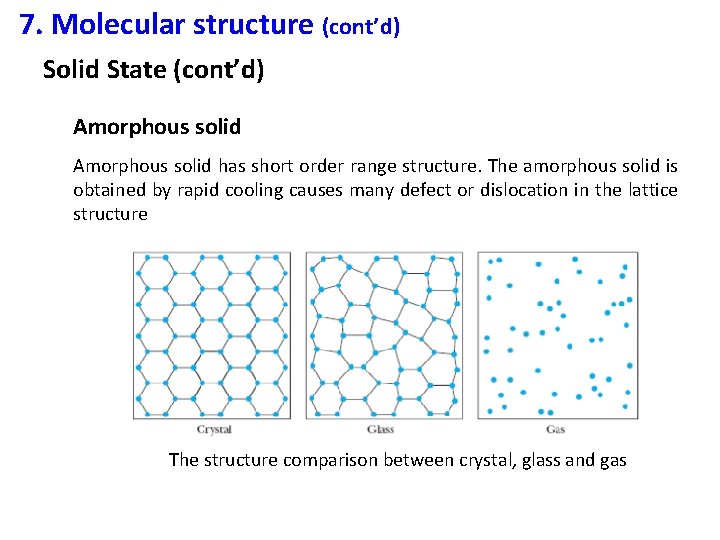
7. Molecular structure (cont’d) Solid State (cont’d) Amorphous solid has short order range structure. The amorphous solid is obtained by rapid cooling causes many defect or dislocation in the lattice structure The structure comparison between crystal, glass and gas
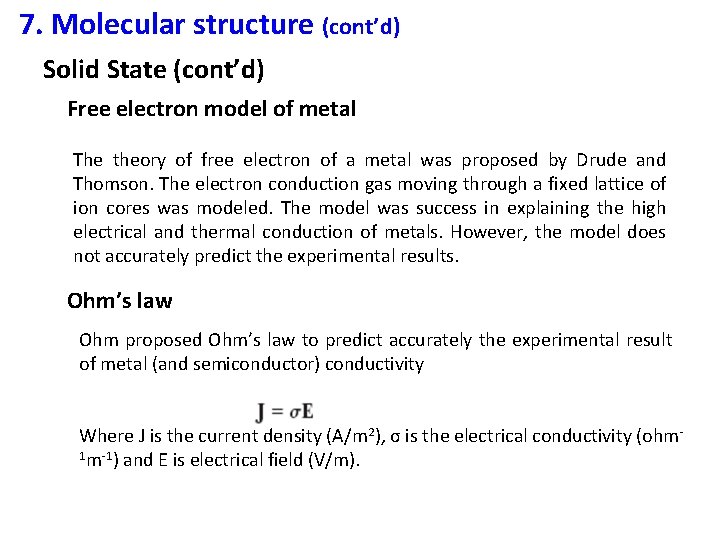
7. Molecular structure (cont’d) Solid State (cont’d) Free electron model of metal The theory of free electron of a metal was proposed by Drude and Thomson. The electron conduction gas moving through a fixed lattice of ion cores was modeled. The model was success in explaining the high electrical and thermal conduction of metals. However, the model does not accurately predict the experimental results. Ohm’s law Ohm proposed Ohm’s law to predict accurately the experimental result of metal (and semiconductor) conductivity Where J is the current density (A/m 2), σ is the electrical conductivity (ohm 1 m-1) and E is electrical field (V/m).
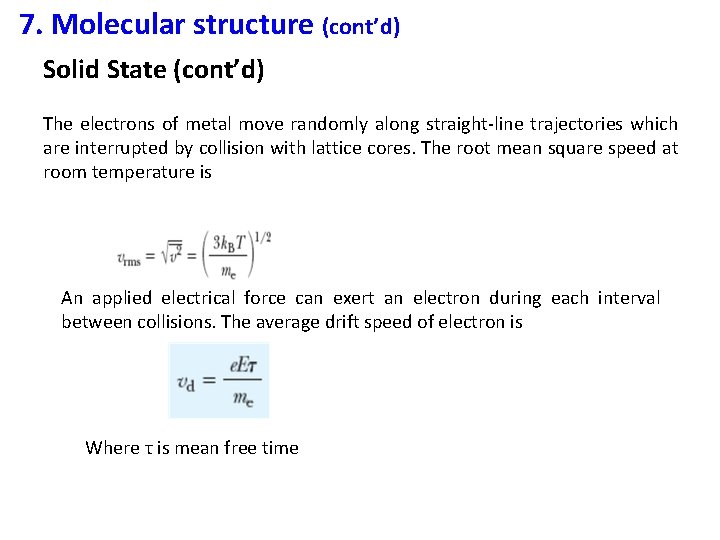
7. Molecular structure (cont’d) Solid State (cont’d) The electrons of metal move randomly along straight-line trajectories which are interrupted by collision with lattice cores. The root mean square speed at room temperature is An applied electrical force can exert an electron during each interval between collisions. The average drift speed of electron is Where τ is mean free time
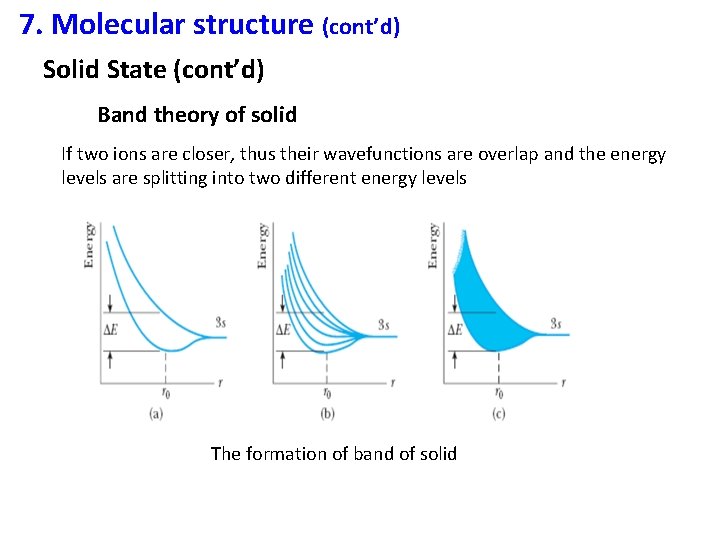
7. Molecular structure (cont’d) Solid State (cont’d) Band theory of solid If two ions are closer, thus their wavefunctions are overlap and the energy levels are splitting into two different energy levels The formation of band of solid
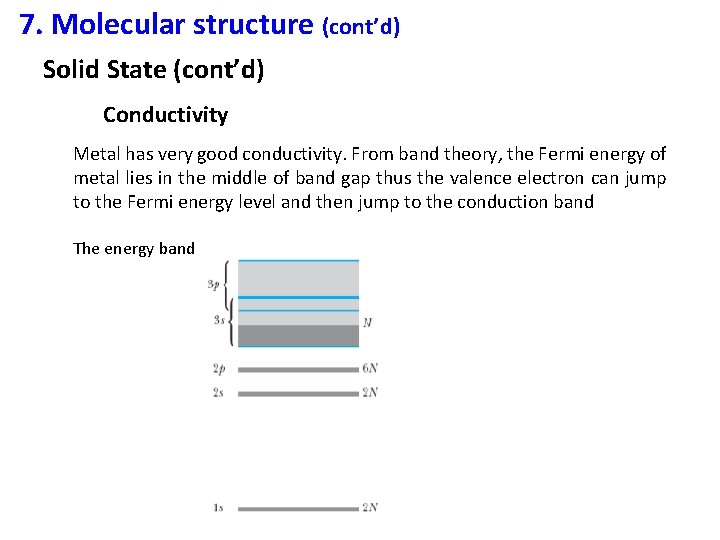
7. Molecular structure (cont’d) Solid State (cont’d) Conductivity Metal has very good conductivity. From band theory, the Fermi energy of metal lies in the middle of band gap thus the valence electron can jump to the Fermi energy level and then jump to the conduction band The energy band
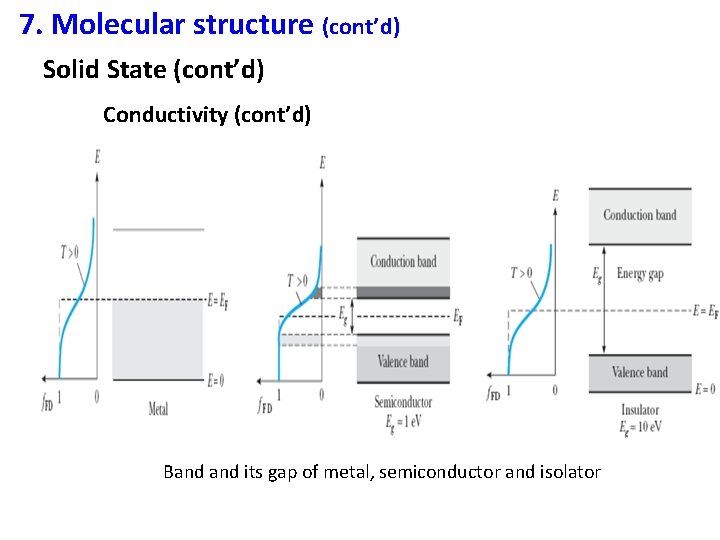
7. Molecular structure (cont’d) Solid State (cont’d) Conductivity (cont’d) Band its gap of metal, semiconductor and isolator
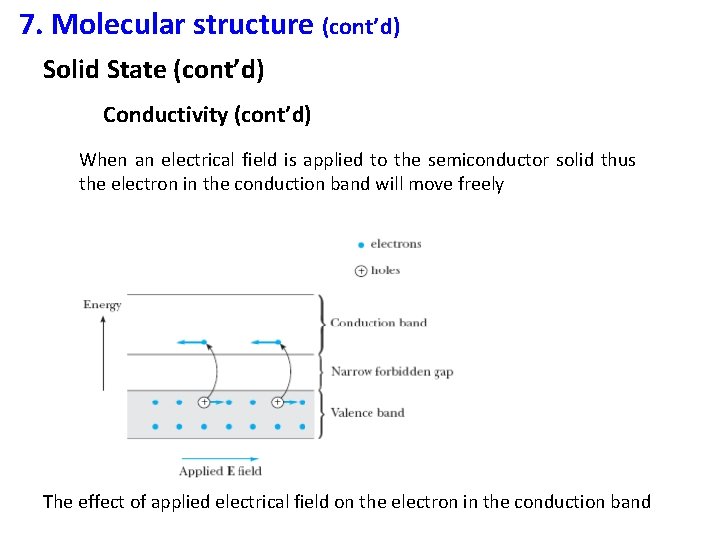
7. Molecular structure (cont’d) Solid State (cont’d) Conductivity (cont’d) When an electrical field is applied to the semiconductor solid thus the electron in the conduction band will move freely The effect of applied electrical field on the electron in the conduction band
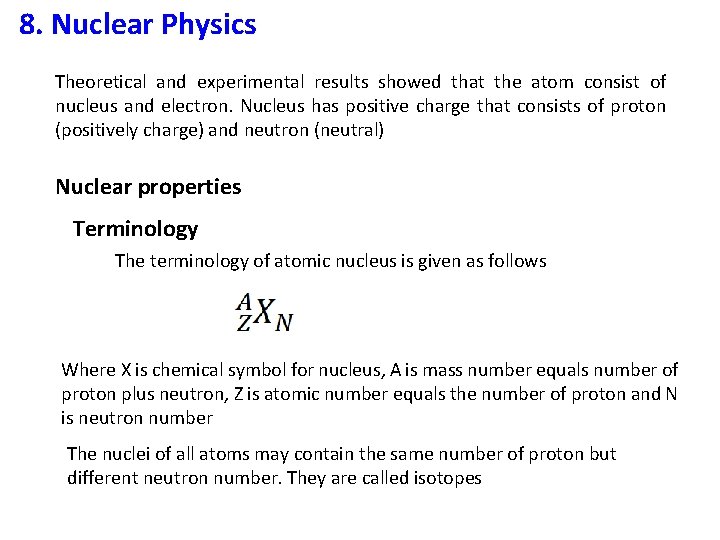
8. Nuclear Physics Theoretical and experimental results showed that the atom consist of nucleus and electron. Nucleus has positive charge that consists of proton (positively charge) and neutron (neutral) Nuclear properties Terminology The terminology of atomic nucleus is given as follows Where X is chemical symbol for nucleus, A is mass number equals number of proton plus neutron, Z is atomic number equals the number of proton and N is neutron number The nuclei of all atoms may contain the same number of proton but different neutron number. They are called isotopes
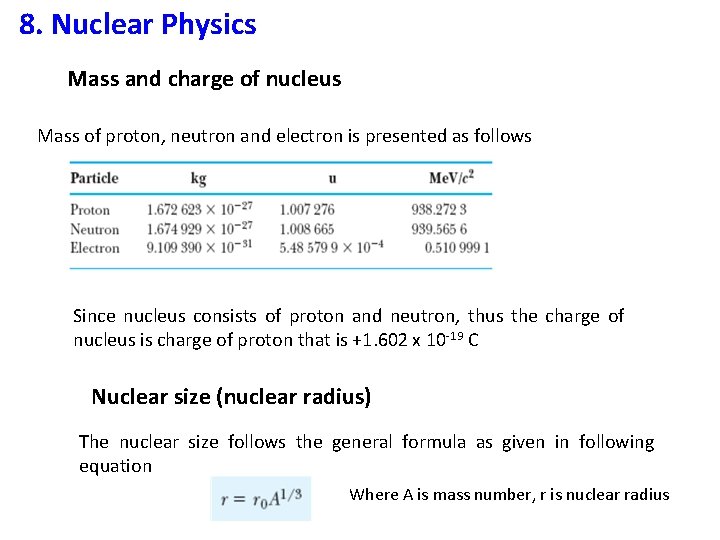
8. Nuclear Physics Mass and charge of nucleus Mass of proton, neutron and electron is presented as follows Since nucleus consists of proton and neutron, thus the charge of nucleus is charge of proton that is +1. 602 x 10 -19 C Nuclear size (nuclear radius) The nuclear size follows the general formula as given in following equation Where A is mass number, r is nuclear radius
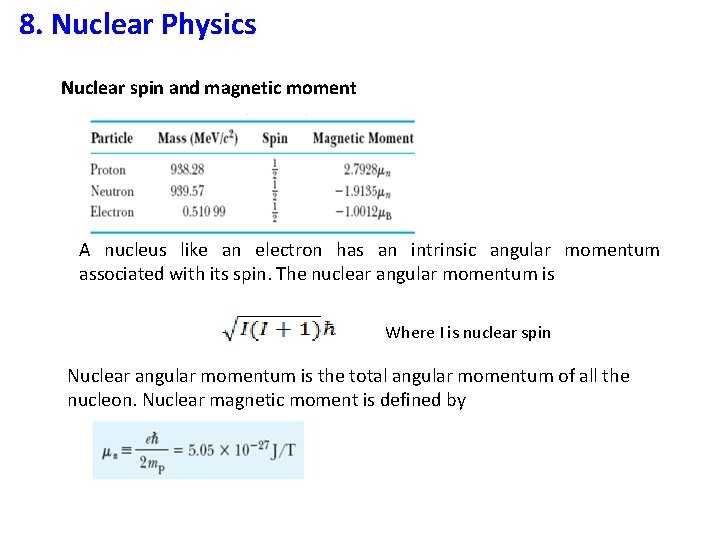
8. Nuclear Physics Nuclear spin and magnetic moment A nucleus like an electron has an intrinsic angular momentum associated with its spin. The nuclear angular momentum is Where I is nuclear spin Nuclear angular momentum is the total angular momentum of all the nucleon. Nuclear magnetic moment is defined by
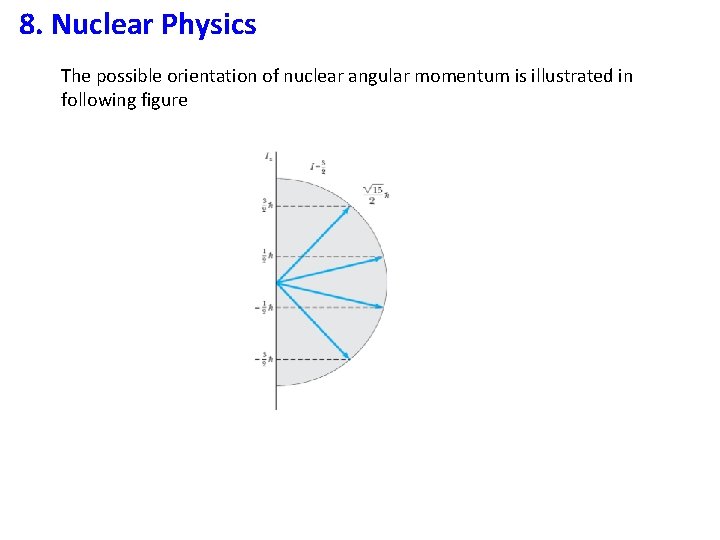
8. Nuclear Physics The possible orientation of nuclear angular momentum is illustrated in following figure
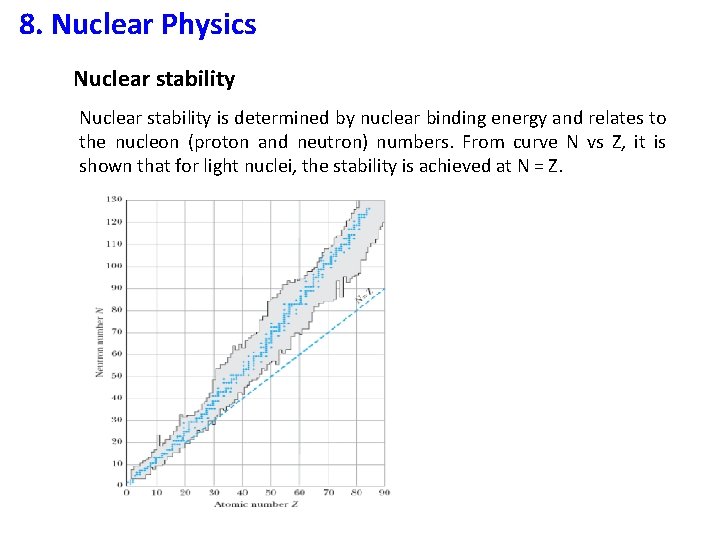
8. Nuclear Physics Nuclear stability is determined by nuclear binding energy and relates to the nucleon (proton and neutron) numbers. From curve N vs Z, it is shown that for light nuclei, the stability is achieved at N = Z.
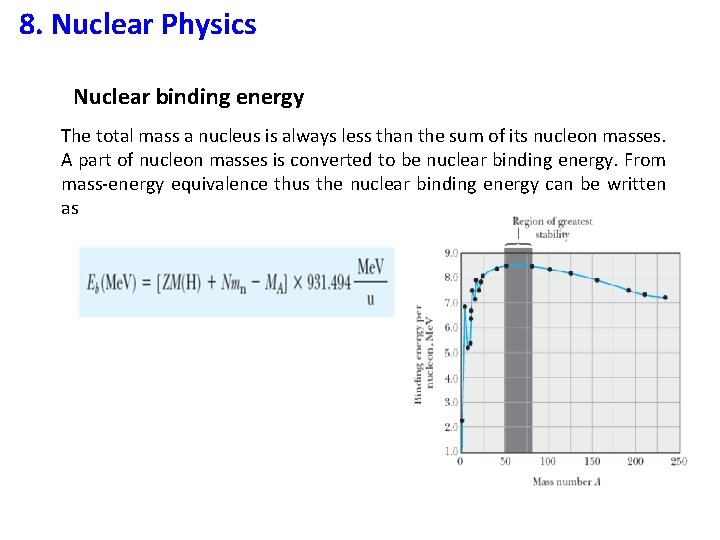
8. Nuclear Physics Nuclear binding energy The total mass a nucleus is always less than the sum of its nucleon masses. A part of nucleon masses is converted to be nuclear binding energy. From mass-energy equivalence thus the nuclear binding energy can be written as
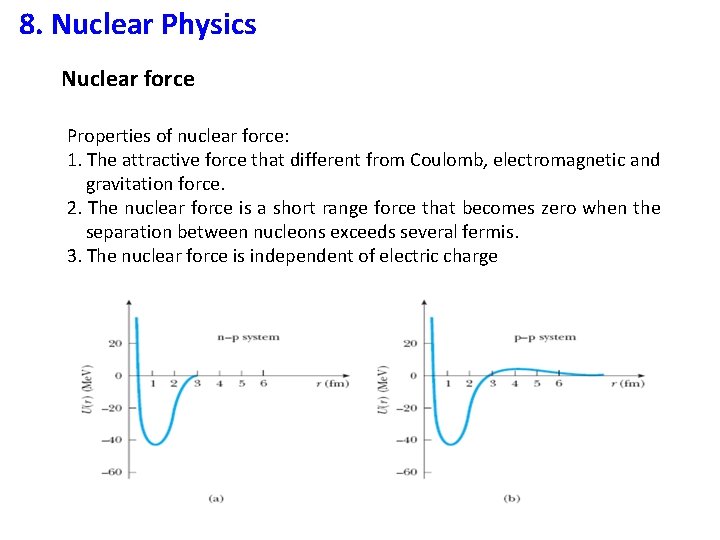
8. Nuclear Physics Nuclear force Properties of nuclear force: 1. The attractive force that different from Coulomb, electromagnetic and gravitation force. 2. The nuclear force is a short range force that becomes zero when the separation between nucleons exceeds several fermis. 3. The nuclear force is independent of electric charge
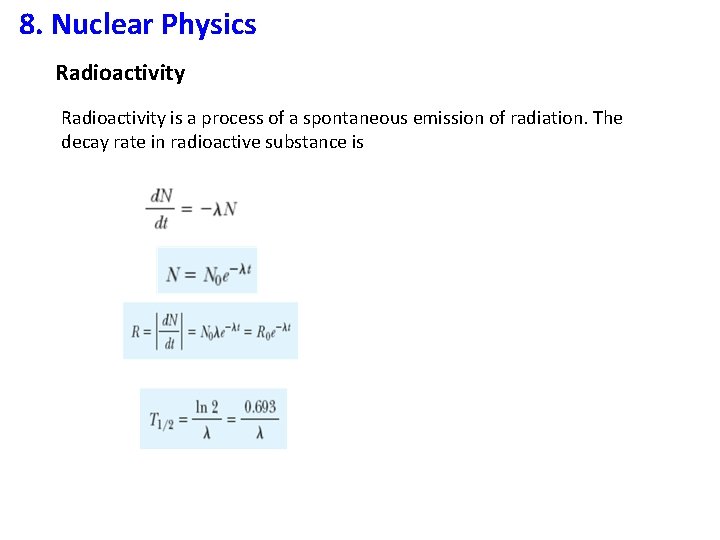
8. Nuclear Physics Radioactivity is a process of a spontaneous emission of radiation. The decay rate in radioactive substance is
Keralastec
Modern physics vs classical physics
University physics with modern physics fifteenth edition
One of my favorite subjects
Bridgeport engineering department
University of bridgeport computer science faculty
Lee kong chian faculty of engineering and science
Florida state university computer science
Ucl computer science modules
Mse iitd
Brown university computer science
Lee kong chian faculty of engineering and science
Iit kanpur physics faculty
Northwestern university computer engineering
Computer science department rutgers
Department of forensic science dc
Ohio department of education science standards
Stanford computer science department
Ubc computer science department
Bhargavi goswami
Computer science department columbia
Princeton
Njit physics department
Department of physics university of tokyo
Warwick physics department
Bhu physics department
Kepler njit
Michigan state astronomy
A pattern of meteorological symbols that represent weather
Forelock in quran
Review of modern physics
Modern physics means
Modern particle physics mark thomson
Modern physics
Modern physics introduction
Modern physics introduction
Modern physics
Modern physics
Social science vs natural science
Branches of natural science diagram
Natural science vs physical science
Applied science vs pure science
Anthropology vs sociology
Science fusion introduction to science and technology
Rule of 70 in population growth
"science author" or "science authors"
Hard and soft science
Science 10 physics review
Physics ib ia examples
Herszon kherson maritime college of merchant marine fleet
Alamo colleges salary schedule
Hahnville high school powerschool
Importance of faculty in higher education
Hubert kairuki memorial university faculty of medicine
Faculty marshall usc advertising csv
Solid thyroid nodule
Penn state neurosurgery
Mercy college adjunct positions
Faculty of medicine nursing and health sciences
Applied medical sciences
Carelli
Faculty of business and economics mendel university in brno
Electrical engineering umd
Factors influencing faculty staff relationship
Czech technical university in prague civil engineering
Faculty 180 ecu
Faculty of engineering shoubra
Singularity university faculty
Faculty of law maastricht
Medical faculty in novi sad dean
Umn faculty dental clinic
Sjsu faculty affairs
Unlv bylaws
Ulm nursing faculty
Symbiosis login page
Faculty model of training
Faculty.washington.edu memory test
Agnes csaki semmelweis
Faculty kutztown carelli
Student faculty ratio for nba
Kfupm clinic
Masaryk university medical faculty
Faculty model of training
Dr veerasamy dallas
Fulbright faculty development program
Faculty of engineering university of porto
Faculty of organizational sciences
Webkredit cuni
Electrotechnical faculty belgrade
Faculty of economics and business debrecen
Kfupm ee faculty
Faculty model of training
Faculty of veterinary medicine cairo university logo
Questrom course evaluations
Faculty model of training
Usf canvas
Canon maastricht university
Faculty of law of the university of zagreb
University of montenegro faculty of law
Civil engineering faculty
University of kragujevac faculty of technical sciences
University of cologne faculty of management
Mycourses rit edu
St.anns college chirala materials
The faculty of observing in any given case
Utar faculty
Tyndale faculty
Leading university founder
University management system dbms project
Electrotechnical faculty belgrade
Etfbl
Faculty of economics and business zagreb
Fitsac
Faculty activity report
Hacettepe university faculty of medicine
Wpi ece tracking sheet
College of charleston faculty salaries
Nyu epilepsy faculty
Bu faculty central
Unt faculty information system
Hijjawi faculty for engineering technology
Pilot dalary
Faculty of veterinary medicine - university of tehran
Semmelweis university faculty of medicine
The faculty
Lebanese university roumieh
Mcgill medicine supporting documents
Willes little flower
Fsu new faculty orientation
Fsu new faculty orientation
B'faculty of humanities agh', b'poland'
York university myfile
Matej bel university in banská bystrica
Faculty introduction speech
Feedback for faculty development programme
Motivated energized and capable faculty
Faculty invitation letter
Clemson university electrical engineering faculty
Faculty of veterinary medicine cairo university
Faculty activity reporting software
Advice for new faculty members
Steal from faculty
Collective and possessive nouns
Feri maribor
Faculty beer menú
Faculty of mechanical engineering thammasat university
Usi faculty
Paws for faculty
Feinberg faculty portal
Chronicle of higher education faculty salaries
Pubh4401
Faculty of engineering orientation
Mendel university faculty of business and economics
Vibrant faculty recruitment
Schematic arrangement of diesel power plant
Studis.fe