Mechanical Ventilation in Status Asthmaticus Dr CC Tsang
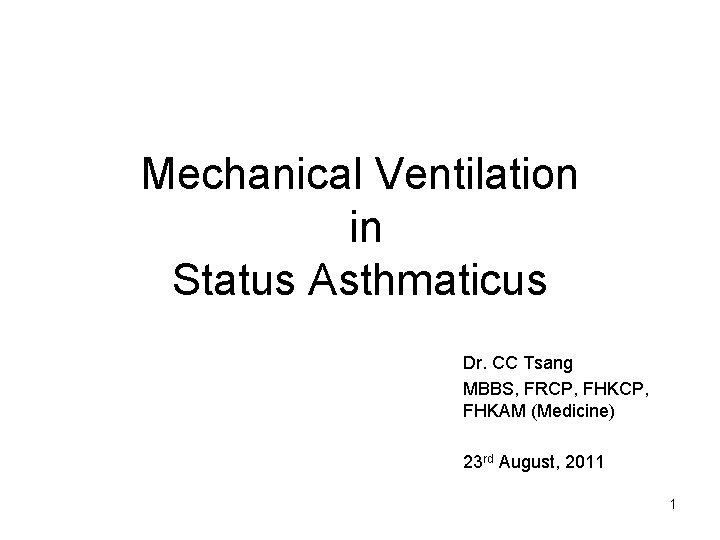
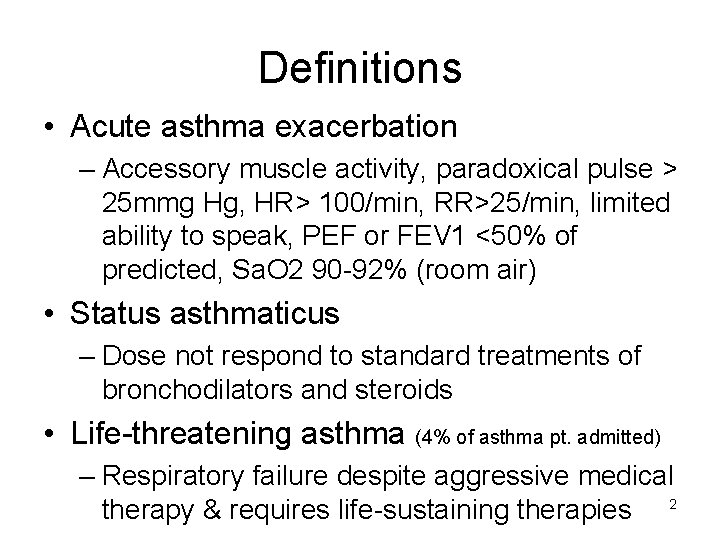
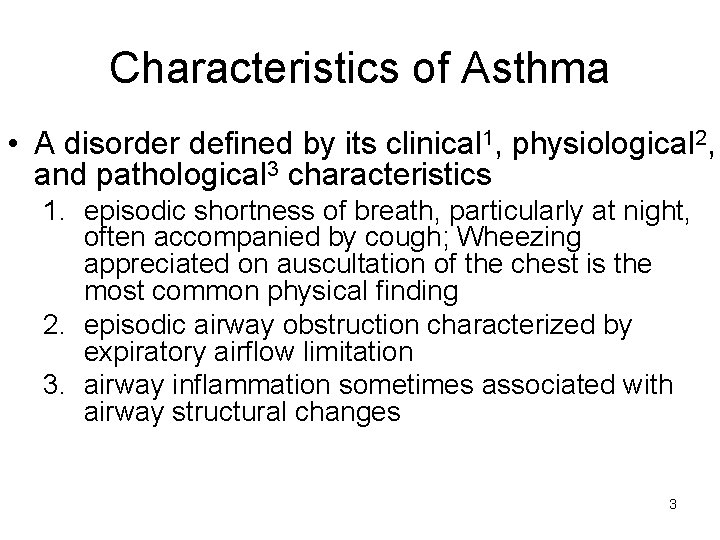
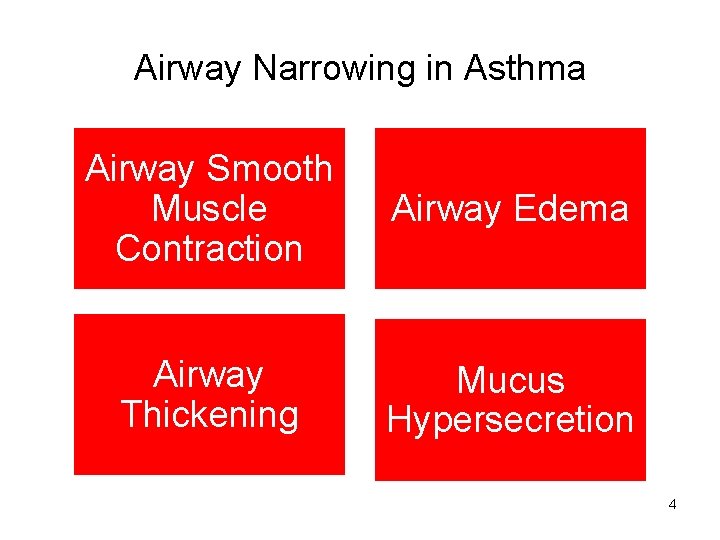
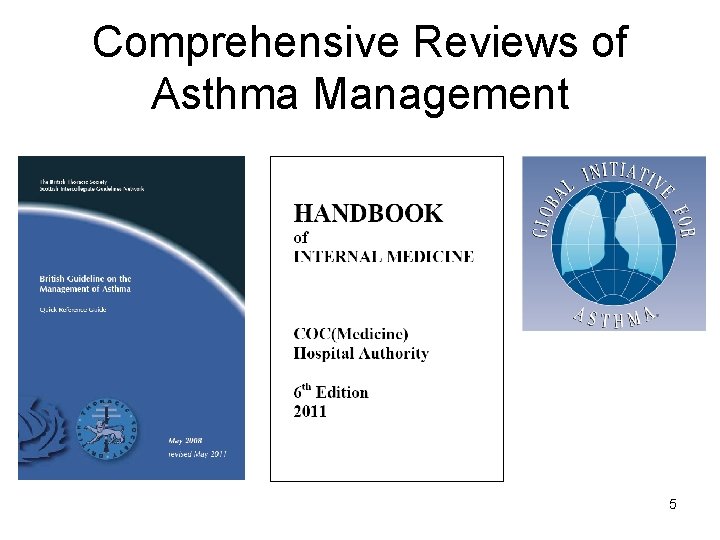
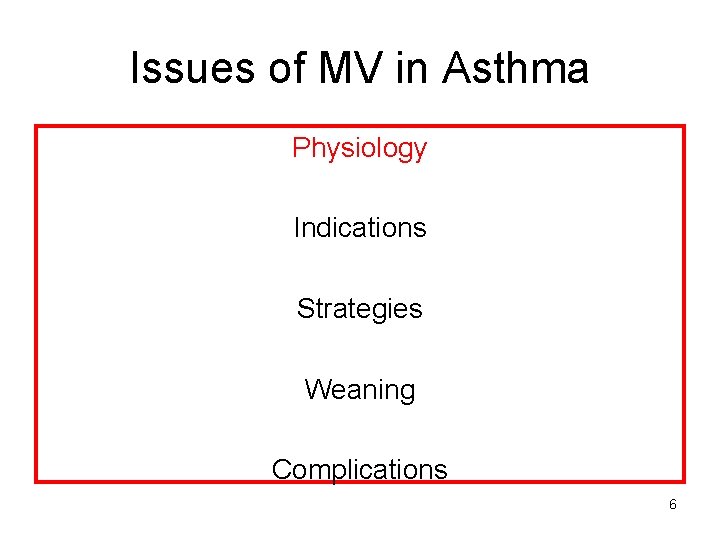
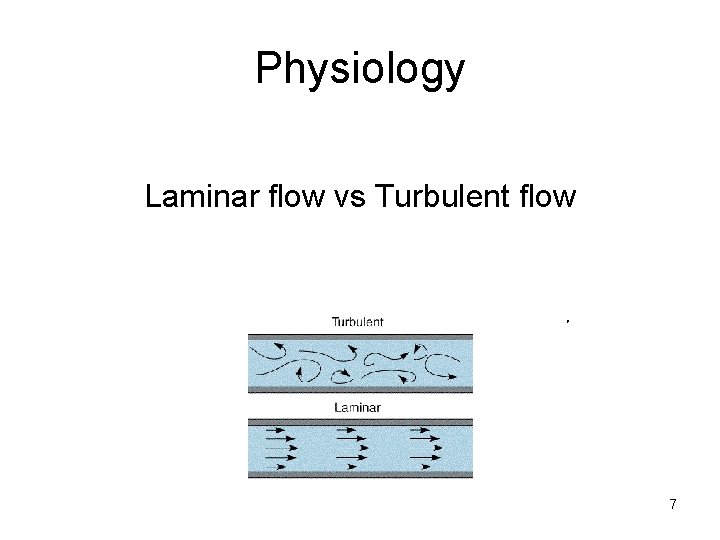
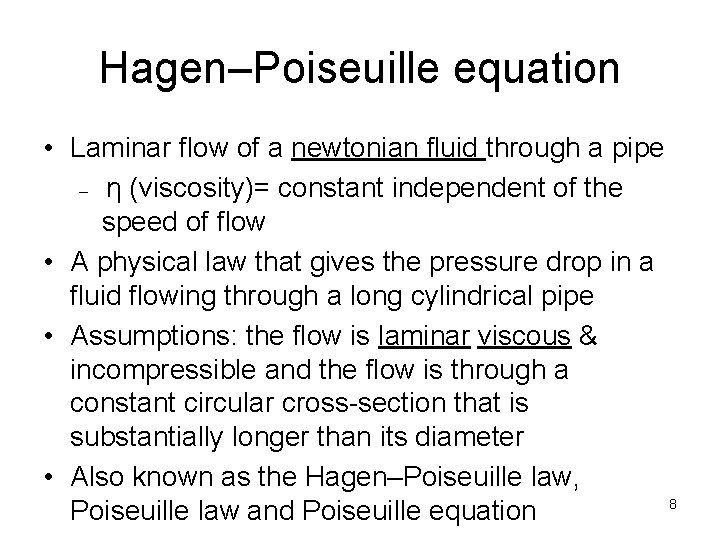
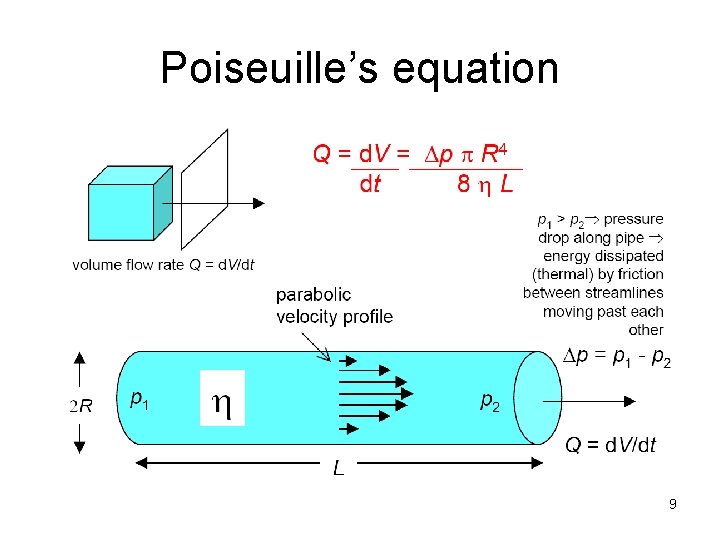
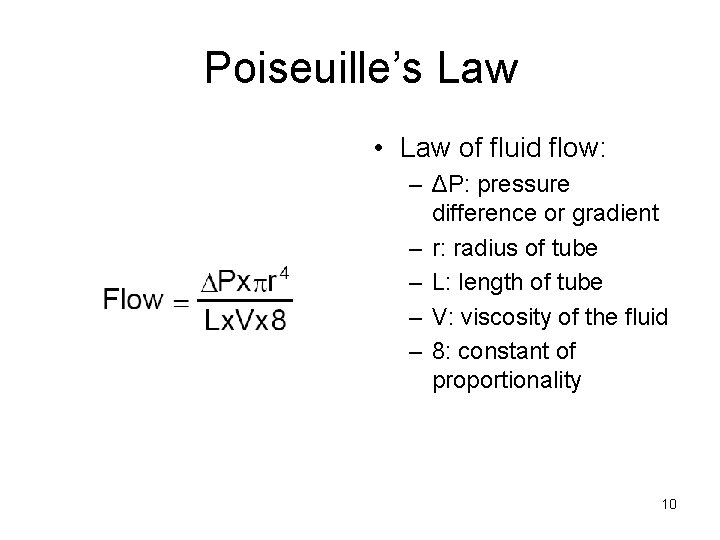
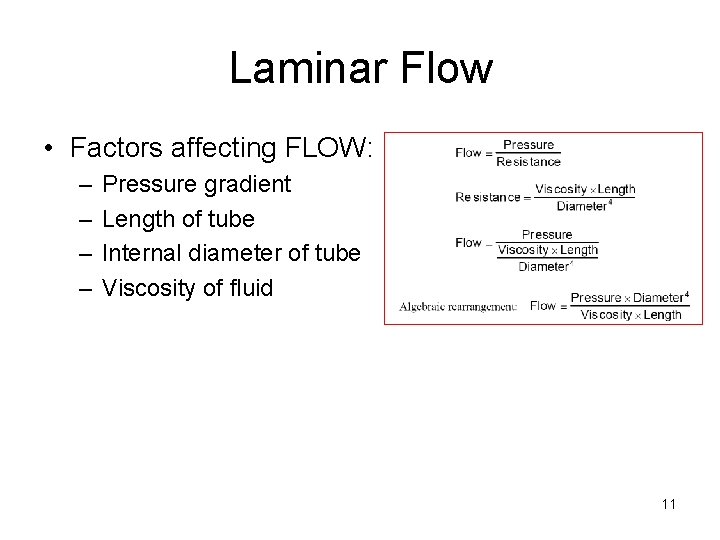
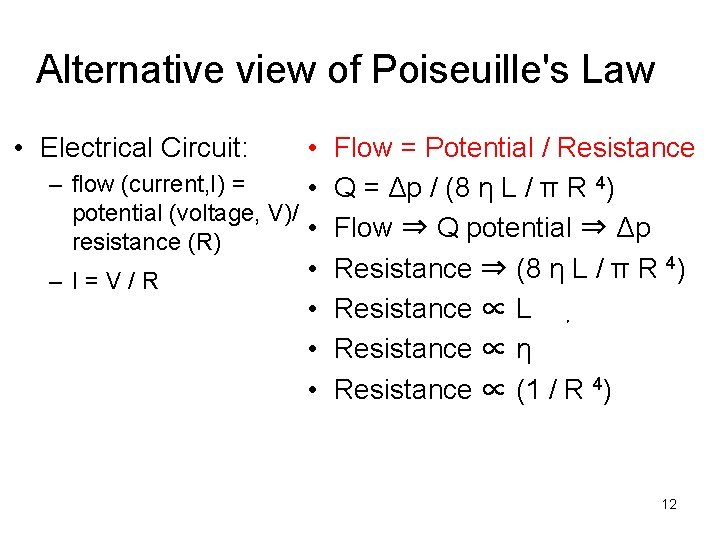
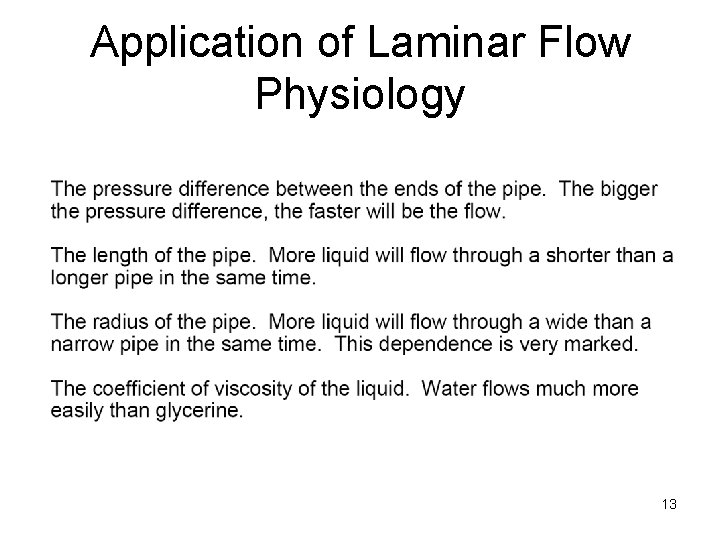
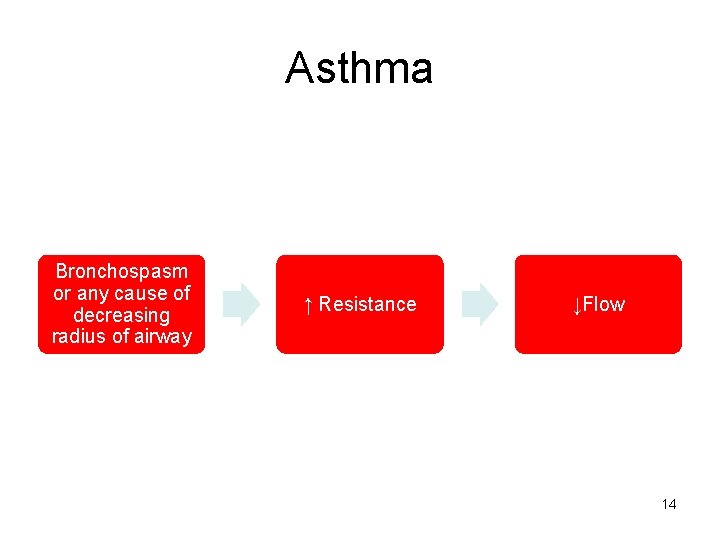
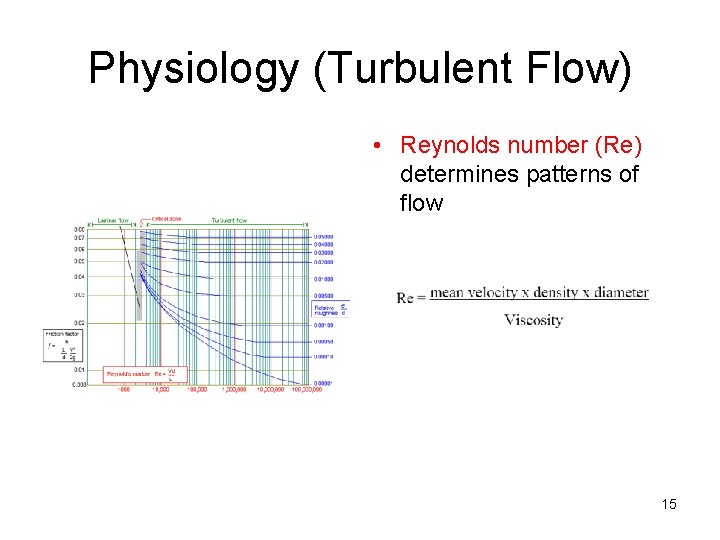
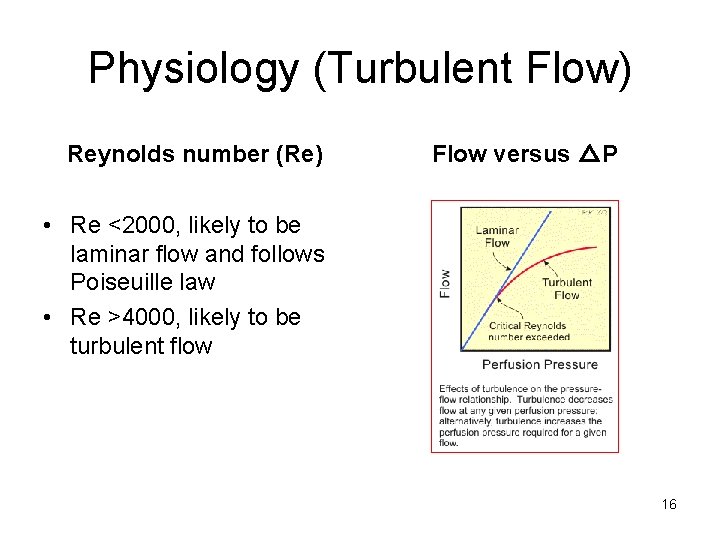
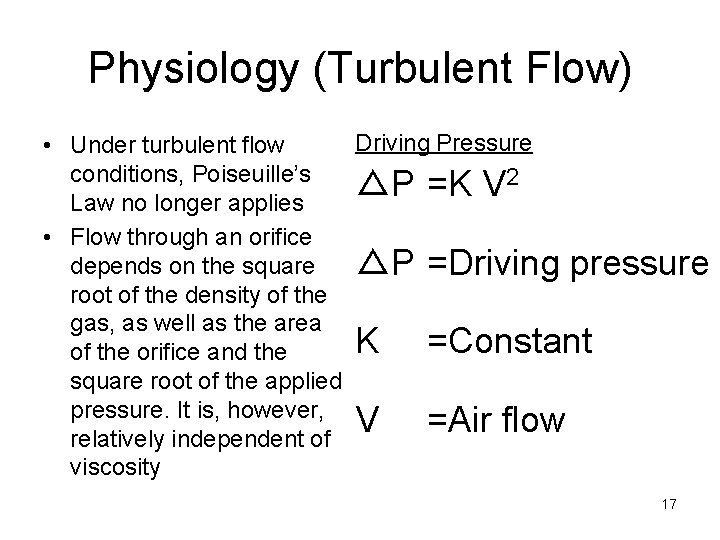
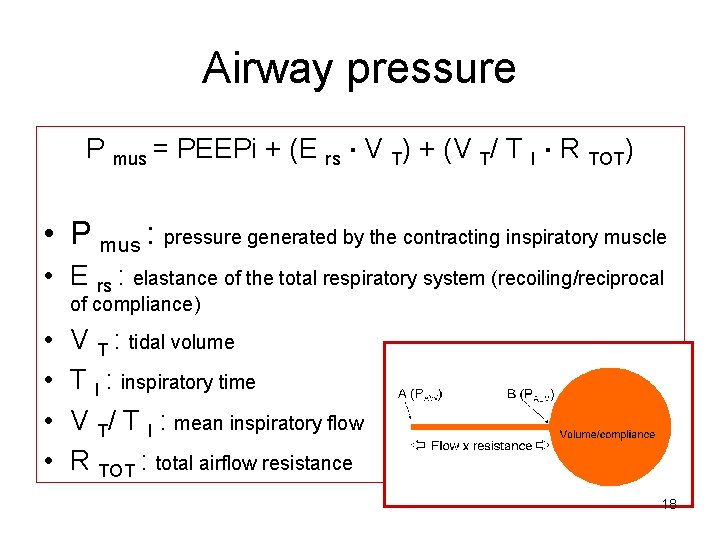
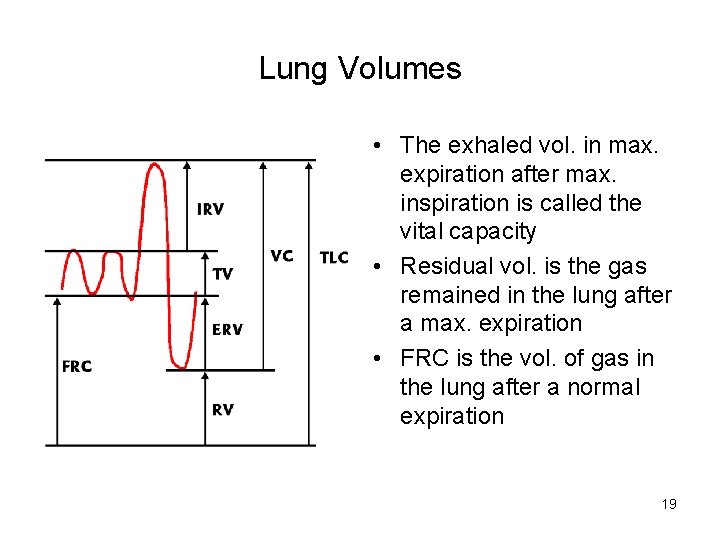
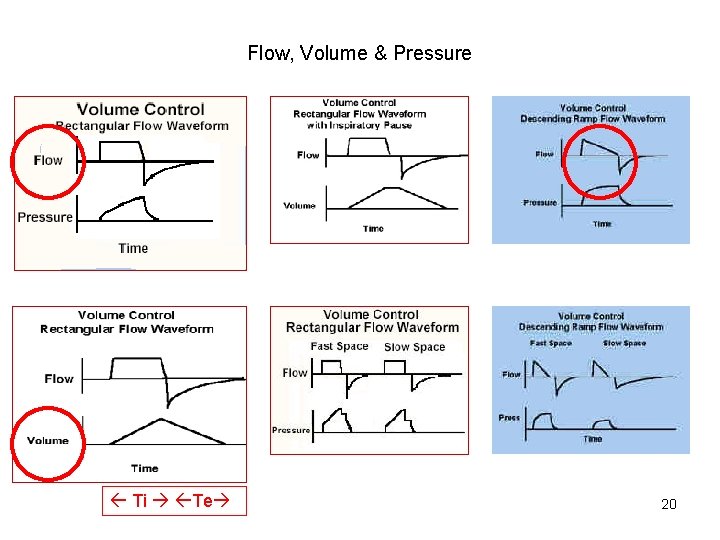
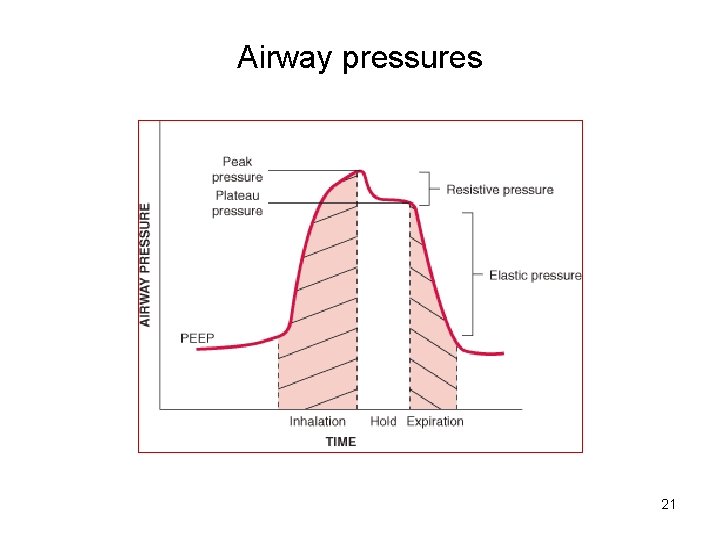
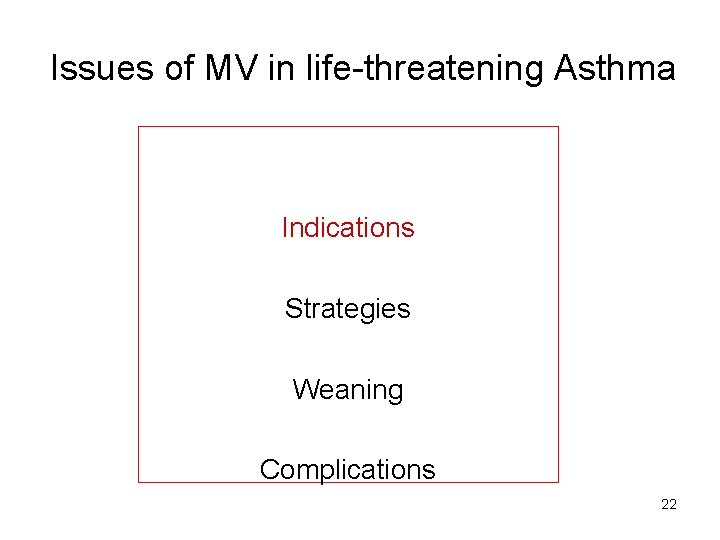
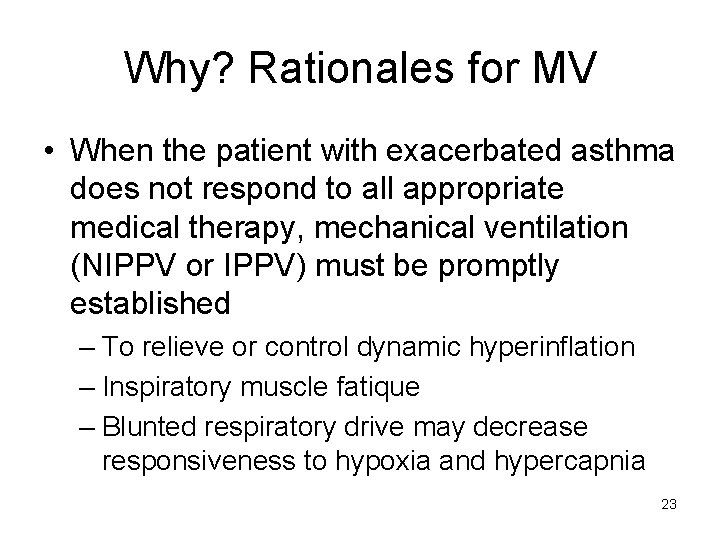
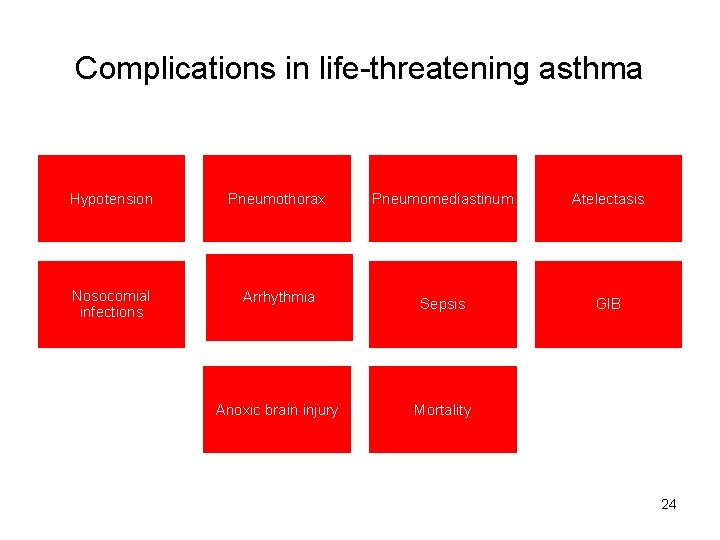
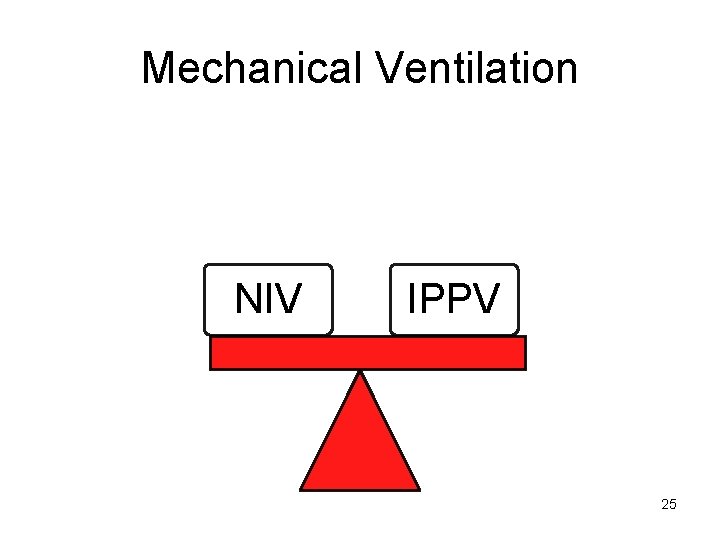
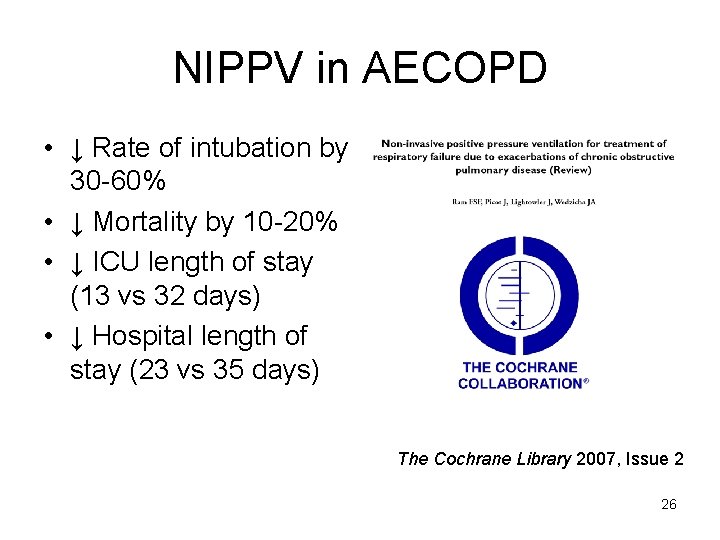
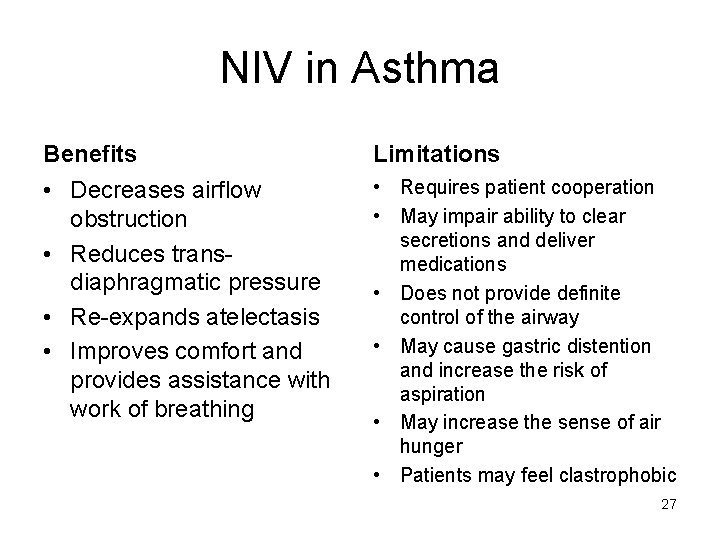
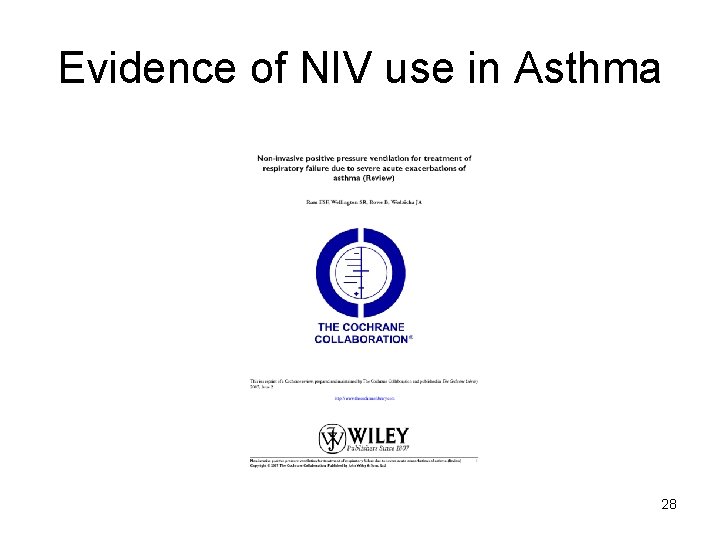
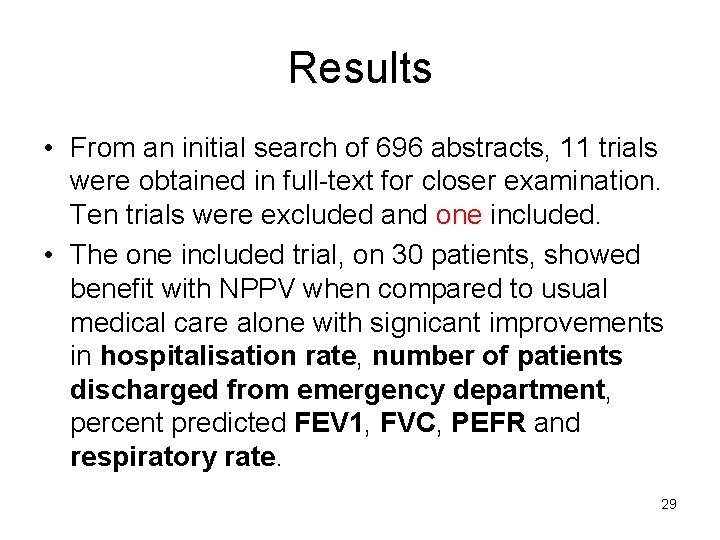
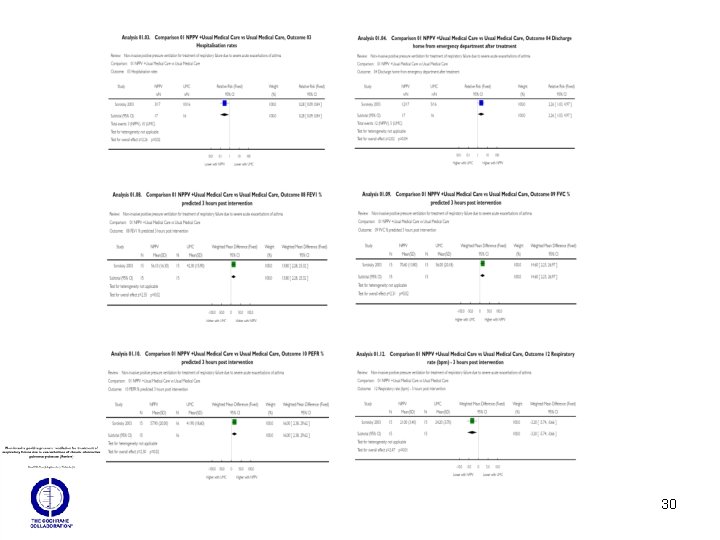
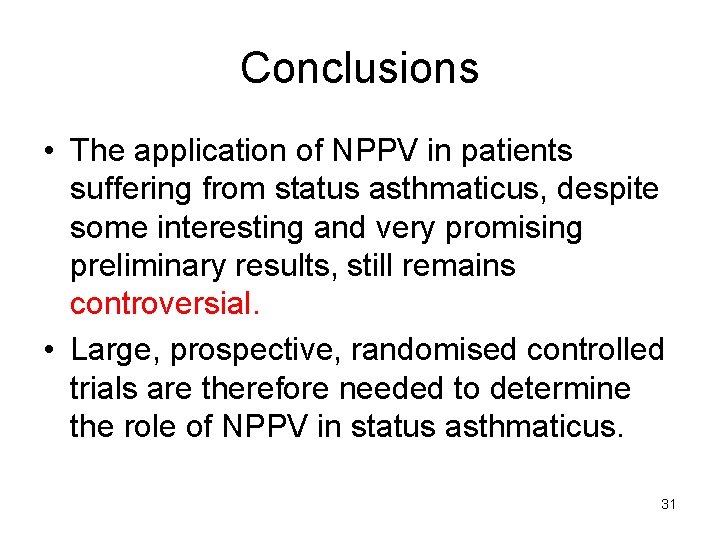
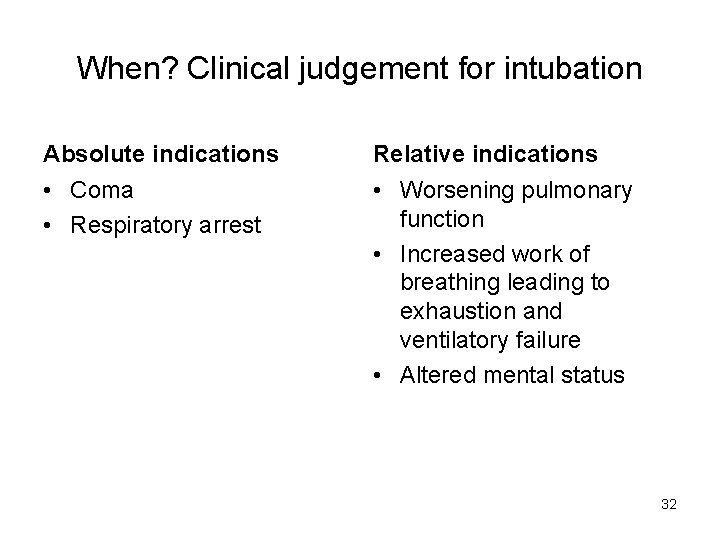
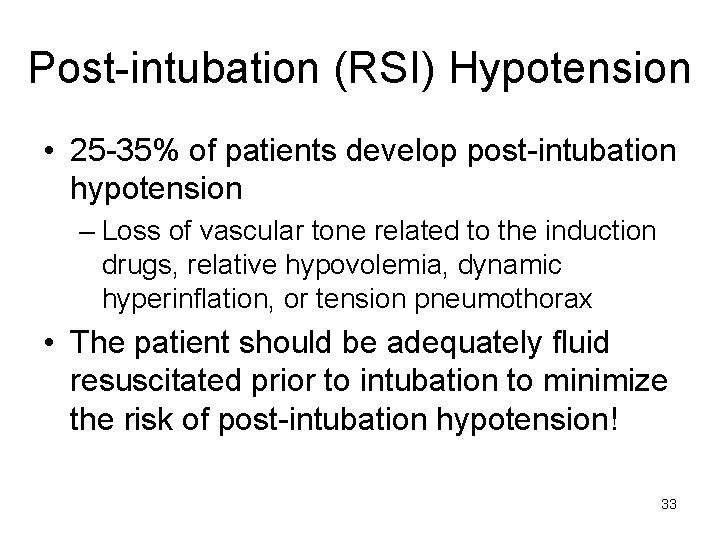
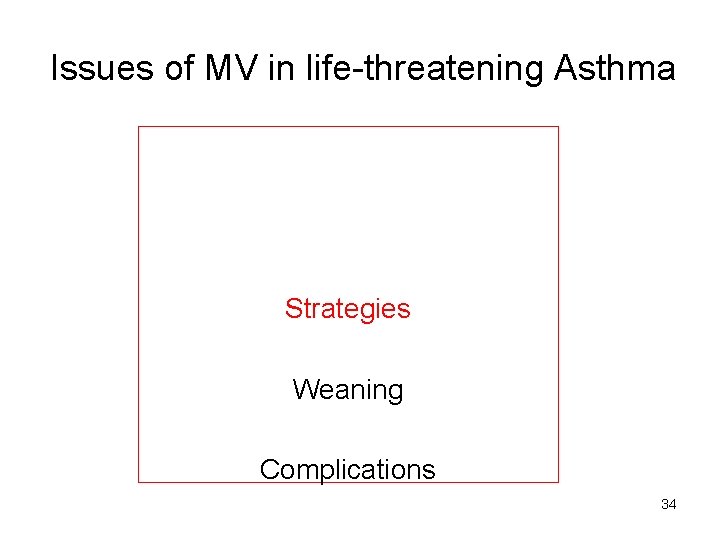
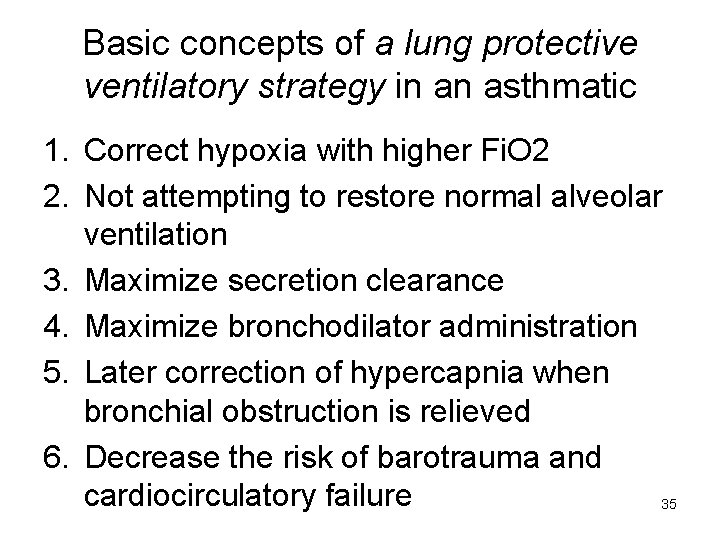
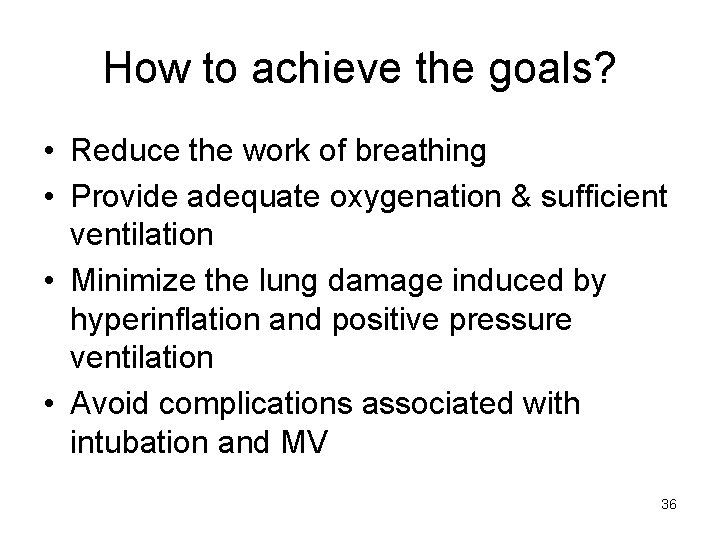
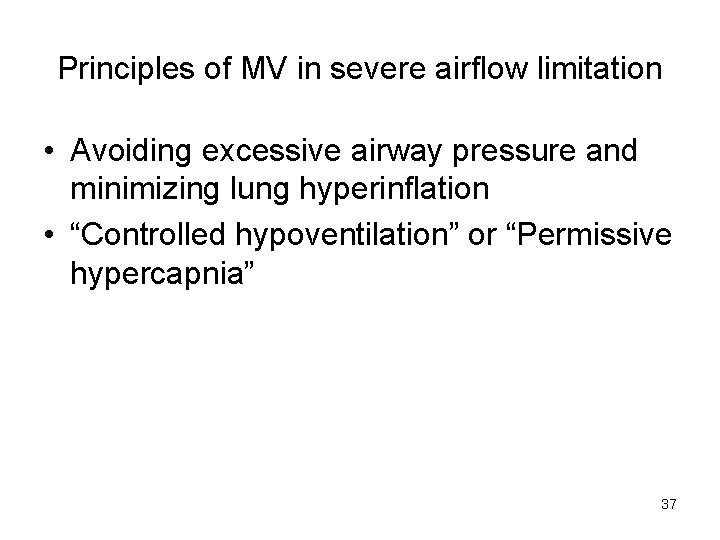
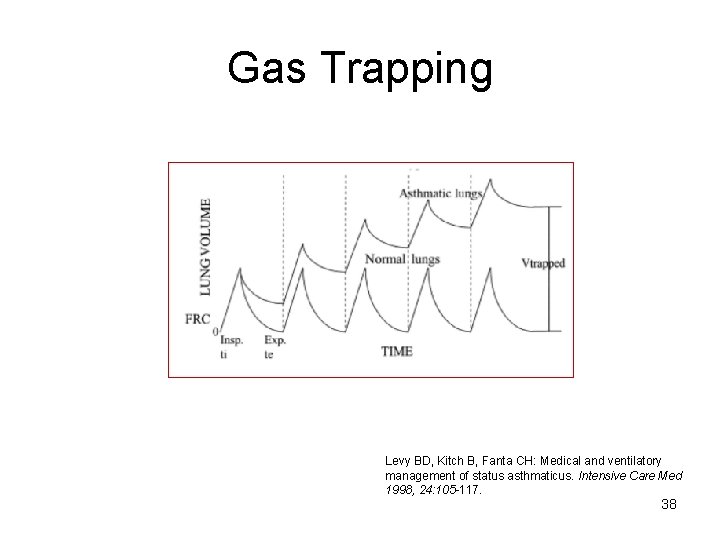
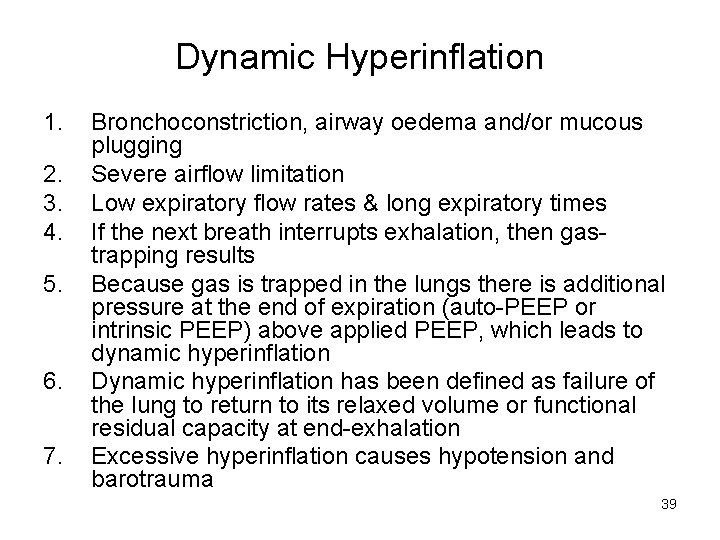
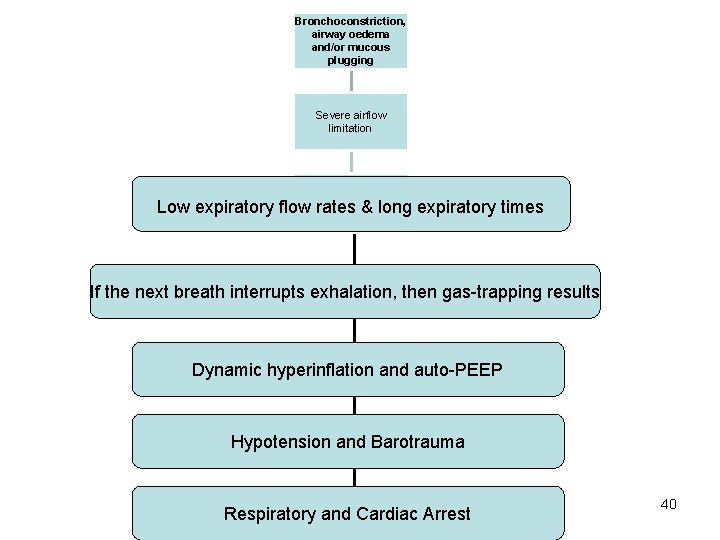
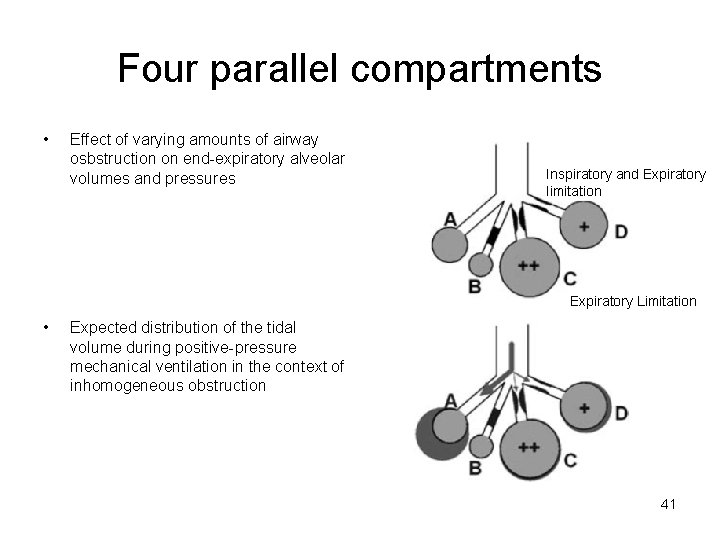
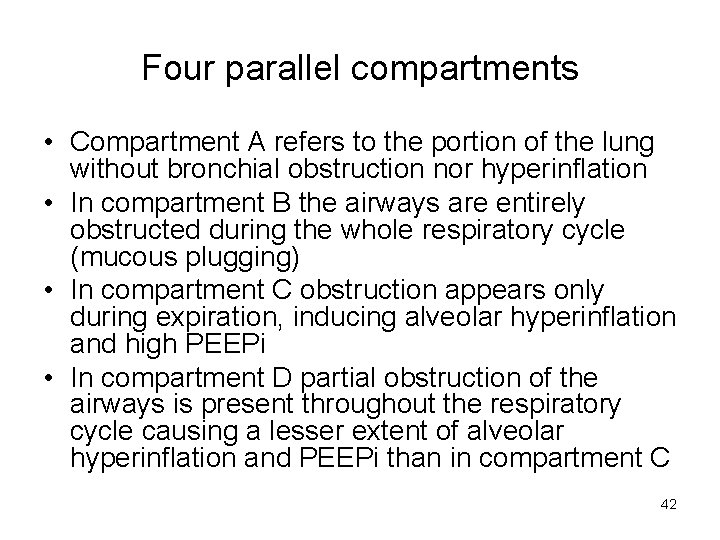
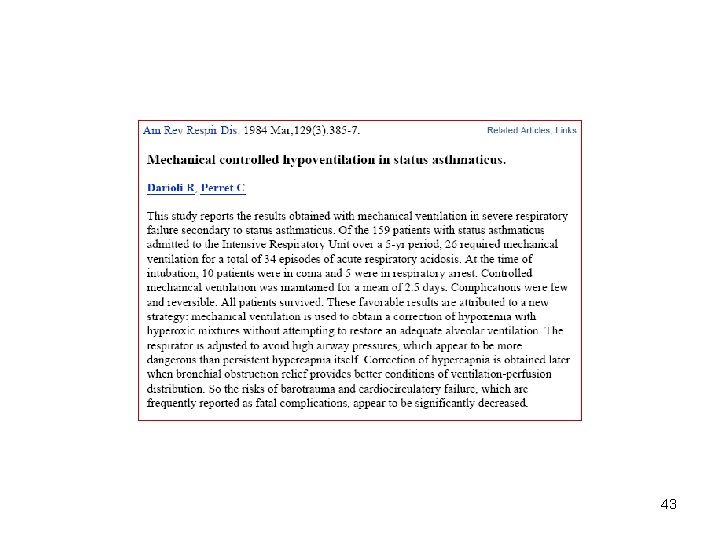
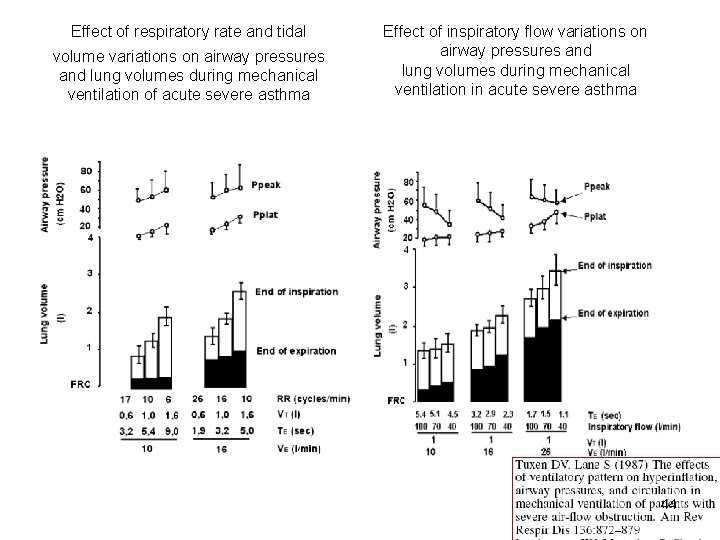
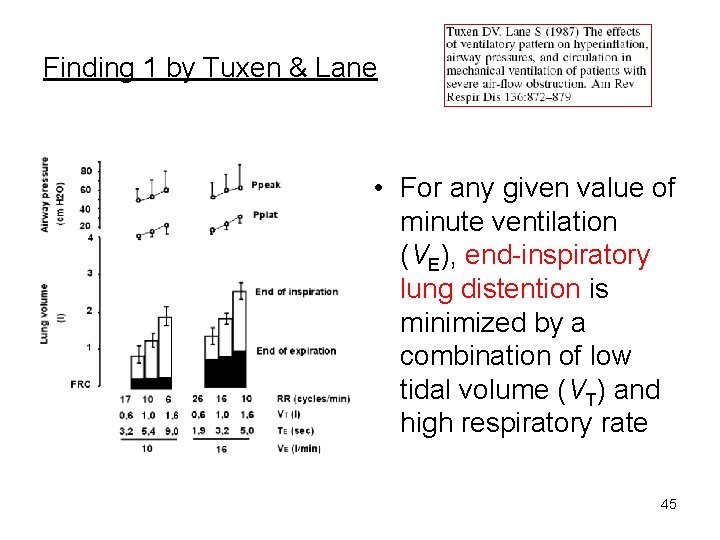
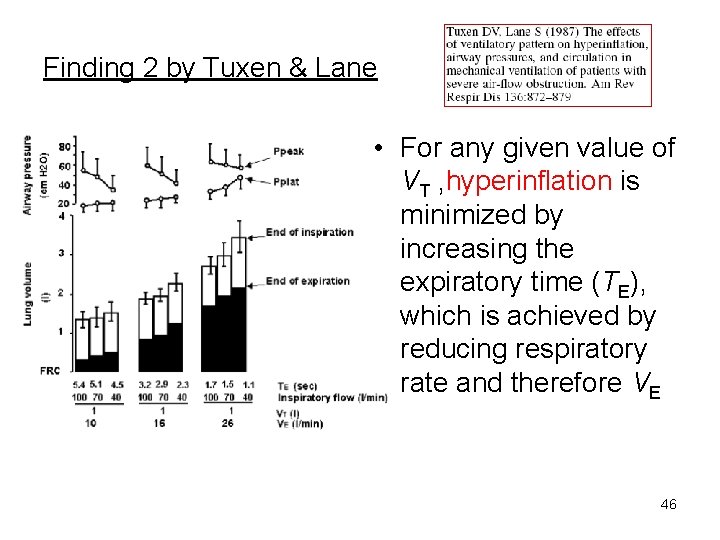
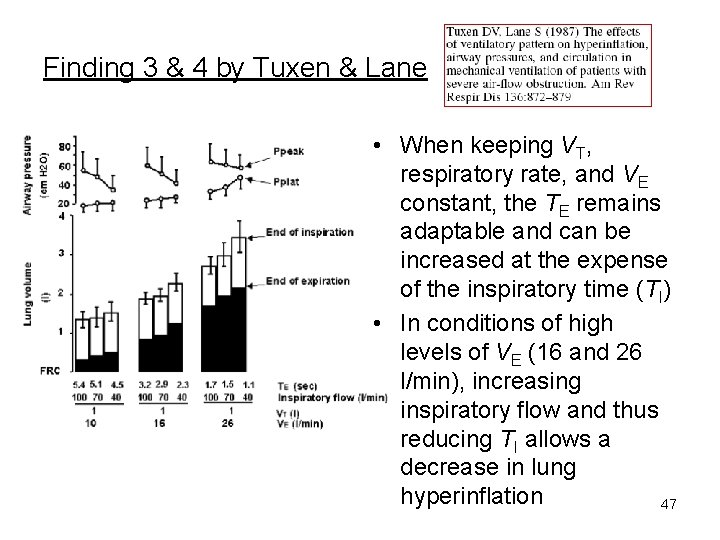
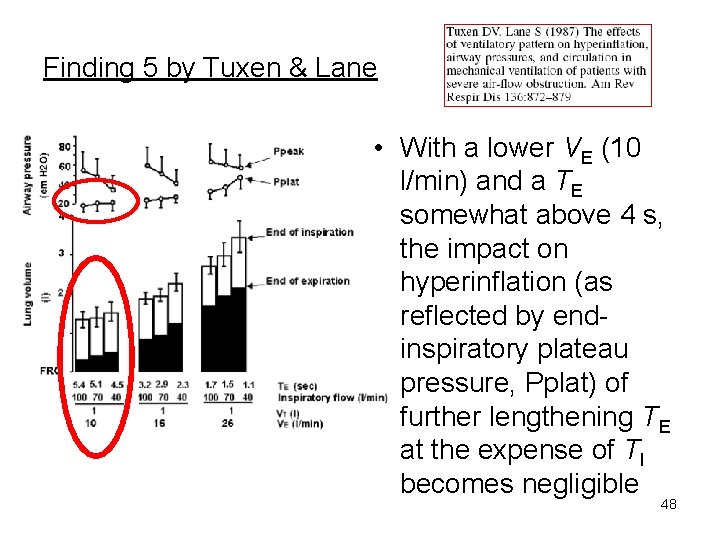
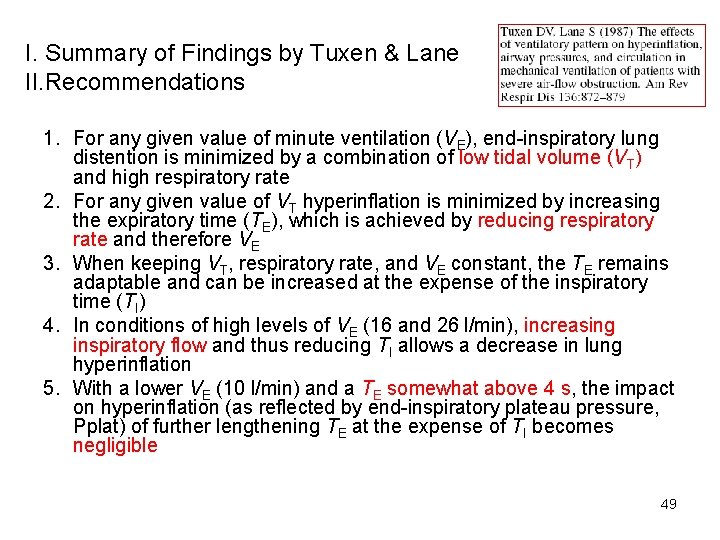
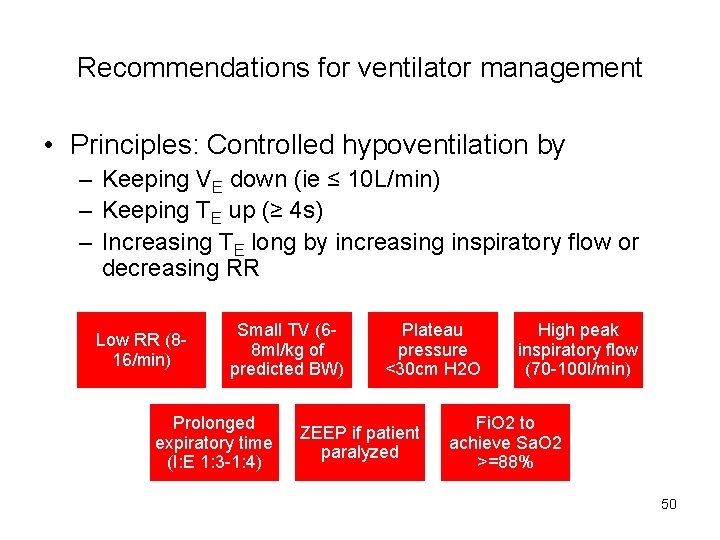
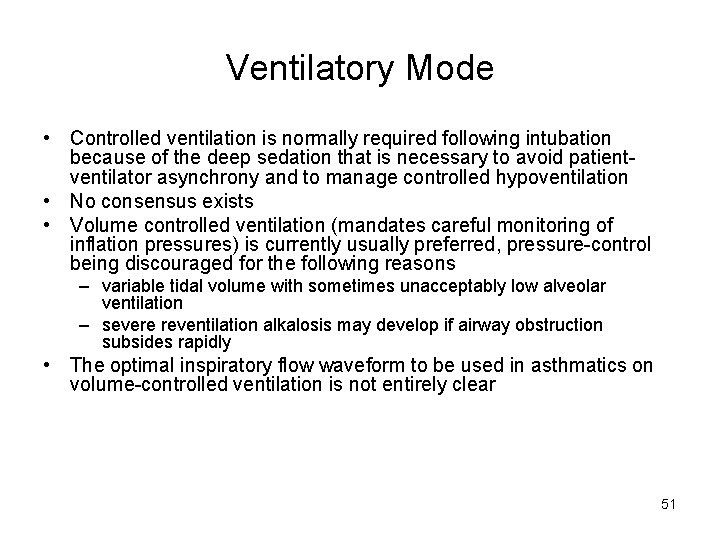
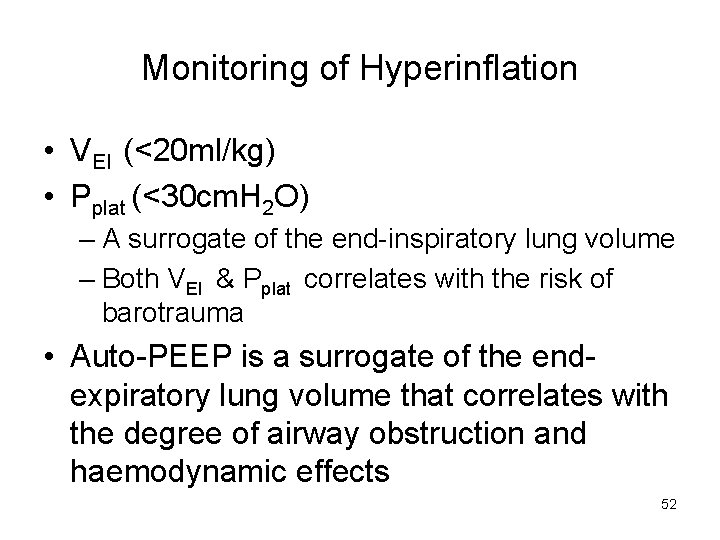
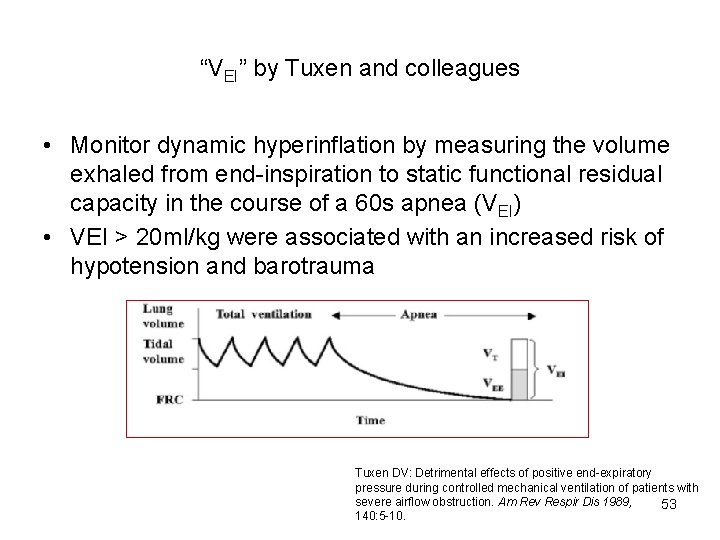
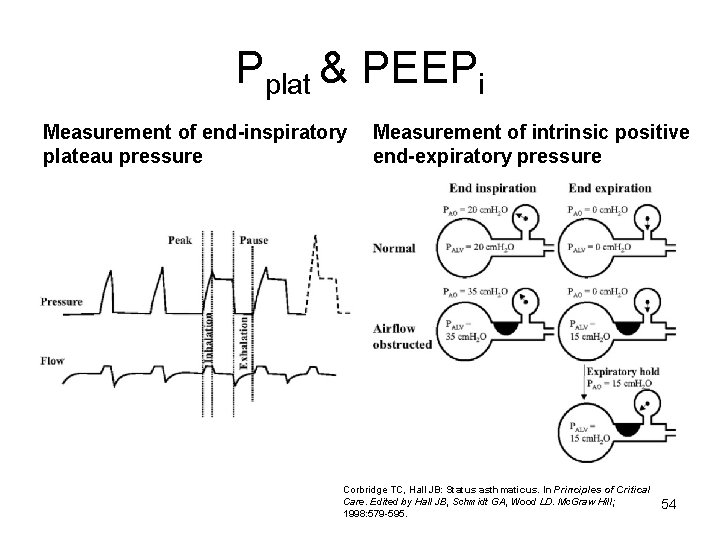
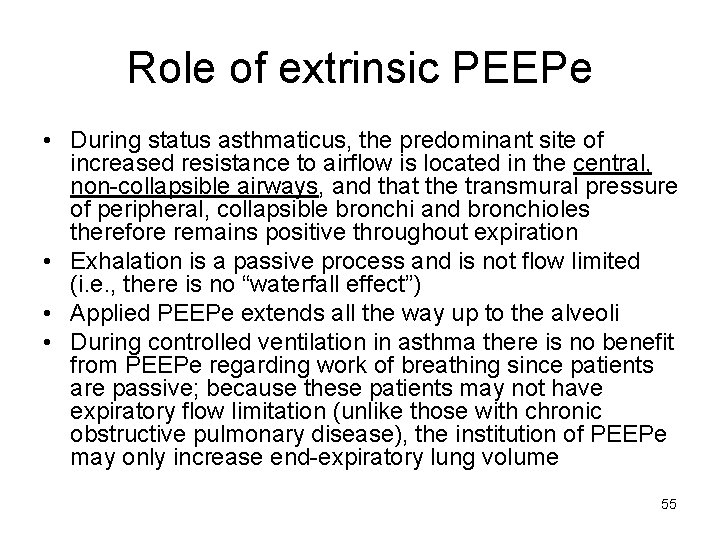
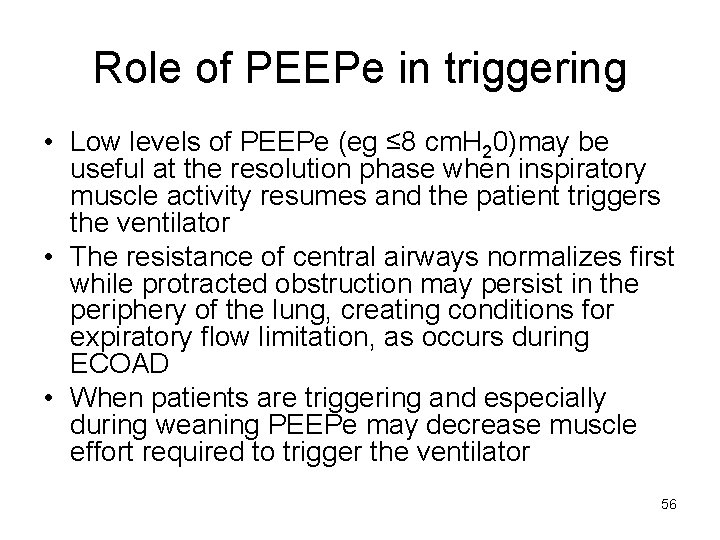
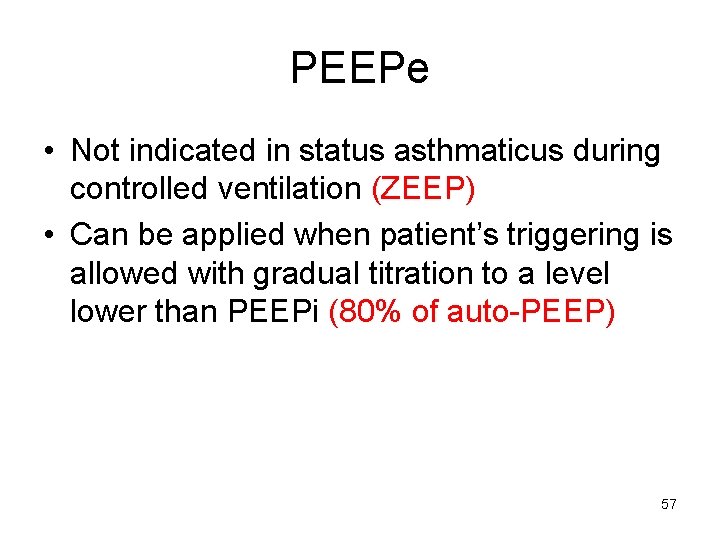
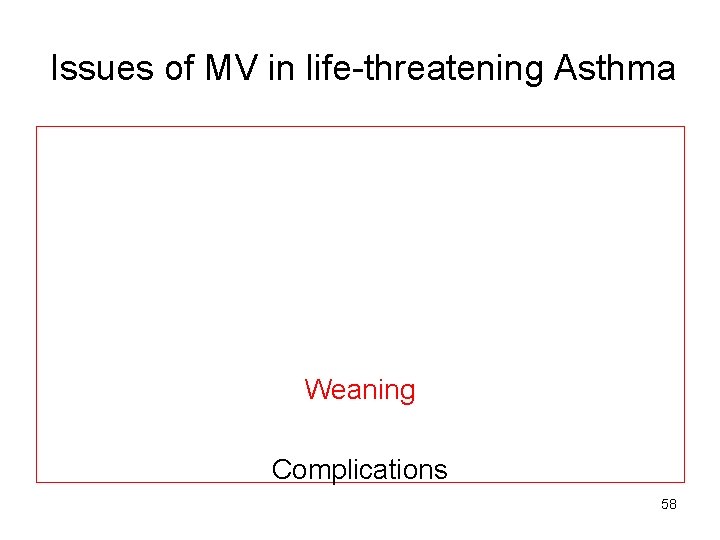
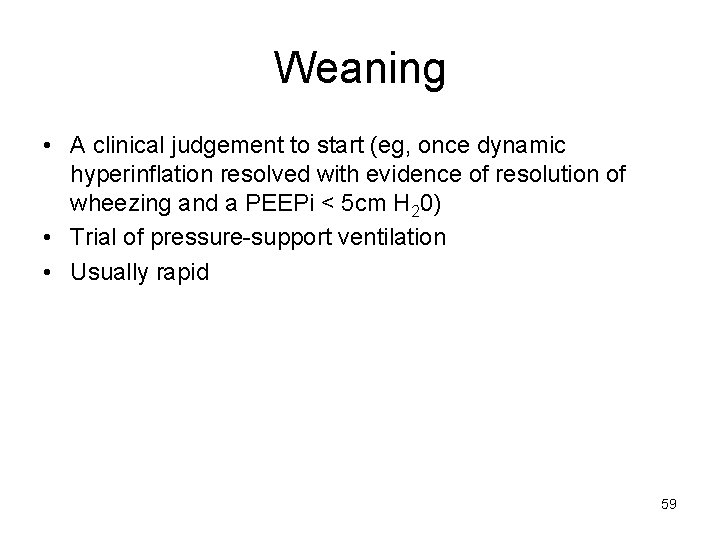
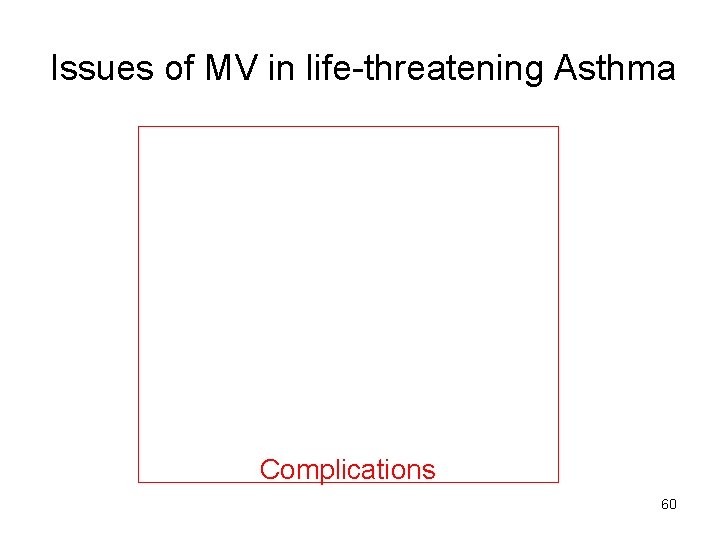
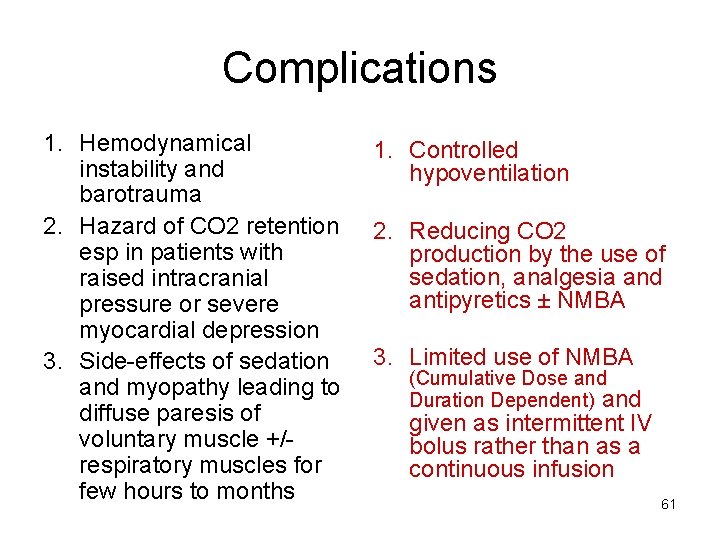
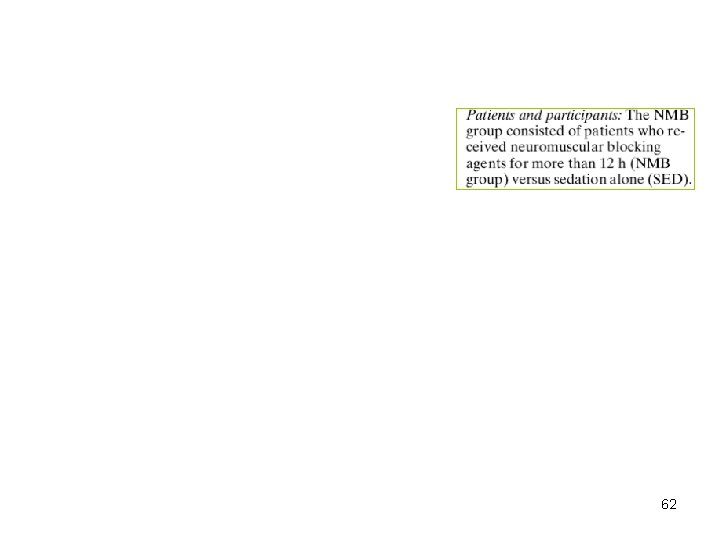
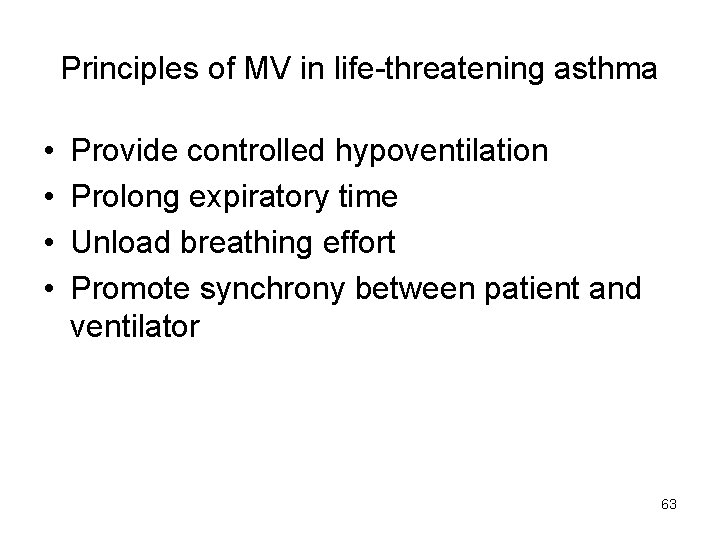
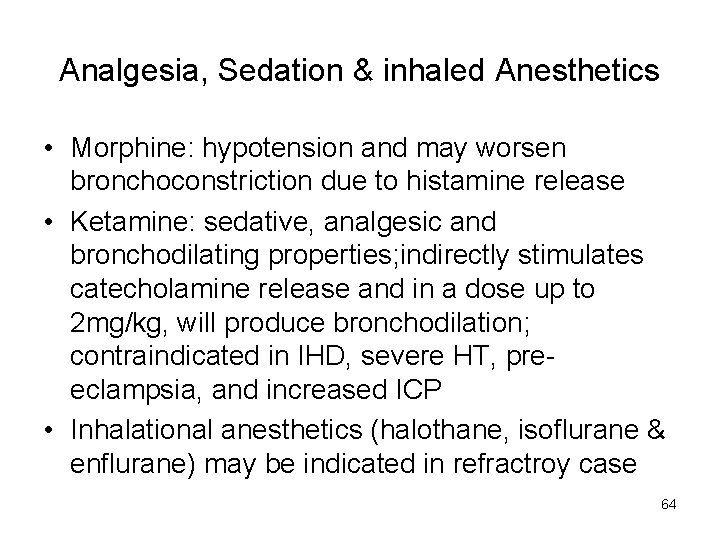
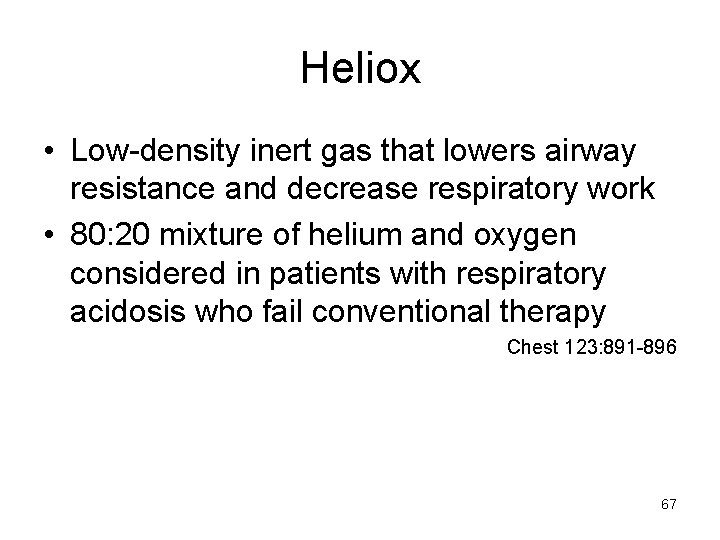
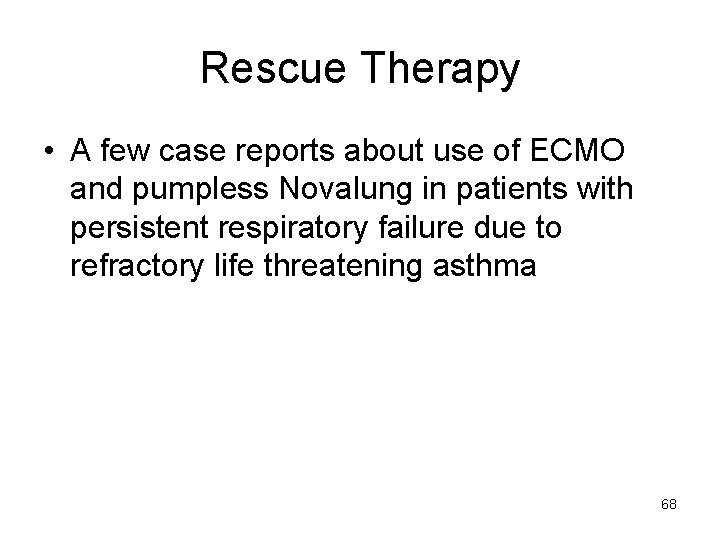
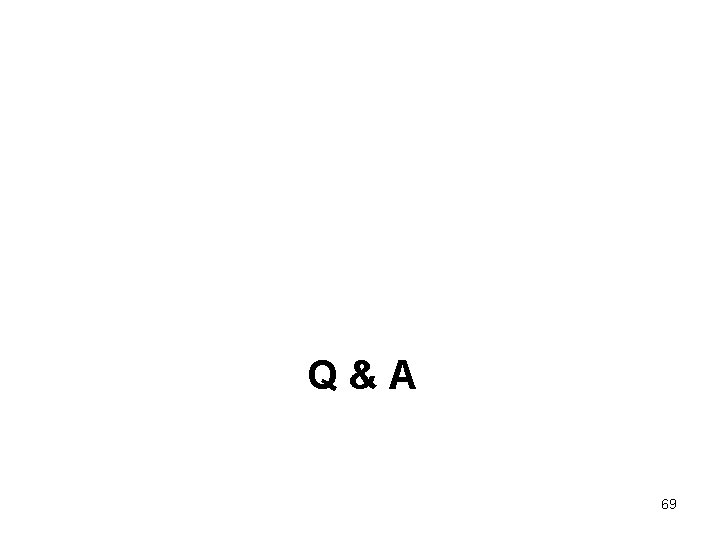
- Slides: 67
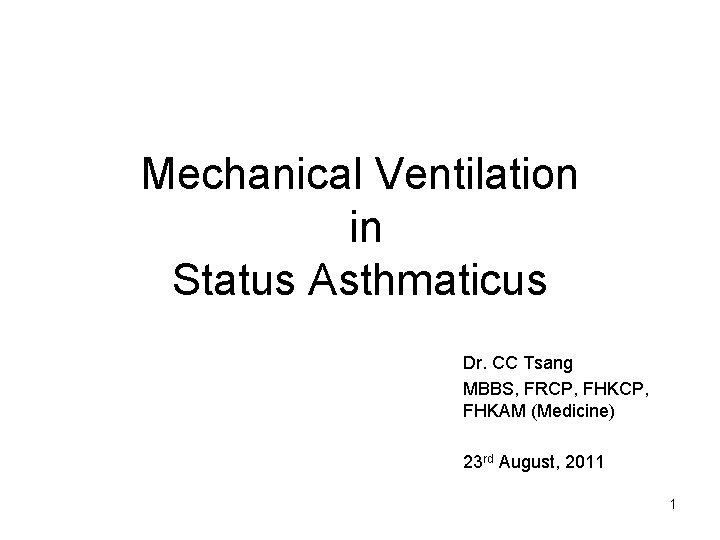
Mechanical Ventilation in Status Asthmaticus Dr. CC Tsang MBBS, FRCP, FHKAM (Medicine) 23 rd August, 2011 1
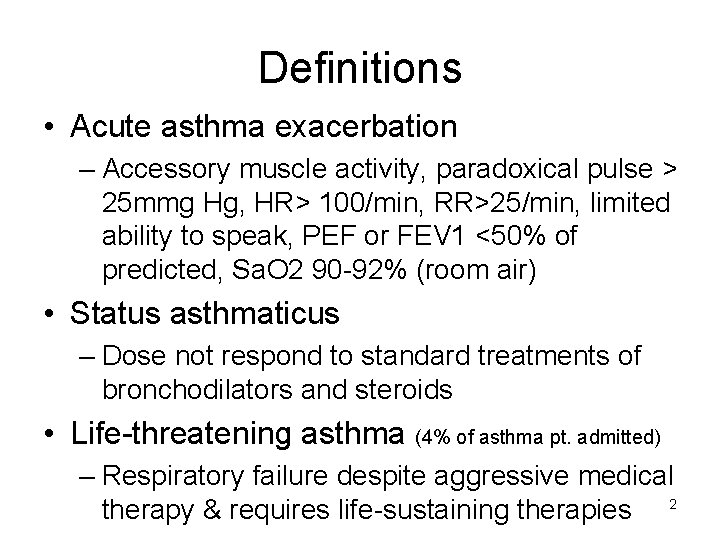
Definitions • Acute asthma exacerbation – Accessory muscle activity, paradoxical pulse > 25 mmg Hg, HR> 100/min, RR>25/min, limited ability to speak, PEF or FEV 1 <50% of predicted, Sa. O 2 90 -92% (room air) • Status asthmaticus – Dose not respond to standard treatments of bronchodilators and steroids • Life-threatening asthma (4% of asthma pt. admitted) – Respiratory failure despite aggressive medical therapy & requires life-sustaining therapies 2
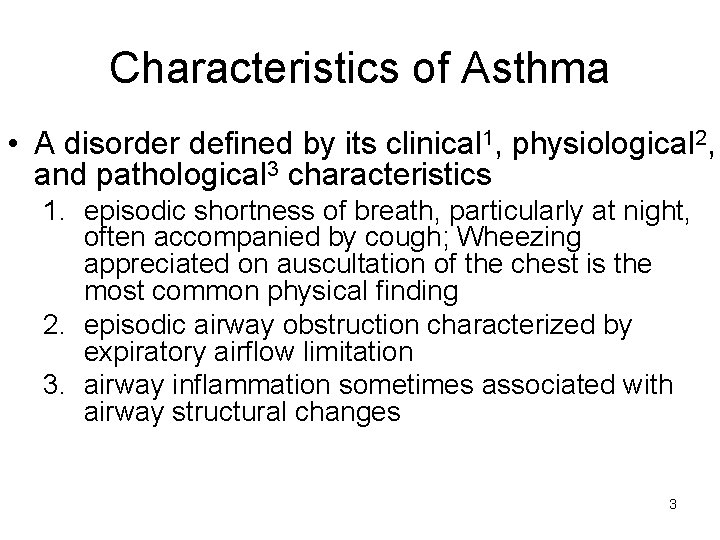
Characteristics of Asthma • A disorder defined by its clinical 1, physiological 2, and pathological 3 characteristics 1. episodic shortness of breath, particularly at night, often accompanied by cough; Wheezing appreciated on auscultation of the chest is the most common physical finding 2. episodic airway obstruction characterized by expiratory airflow limitation 3. airway inflammation sometimes associated with airway structural changes 3
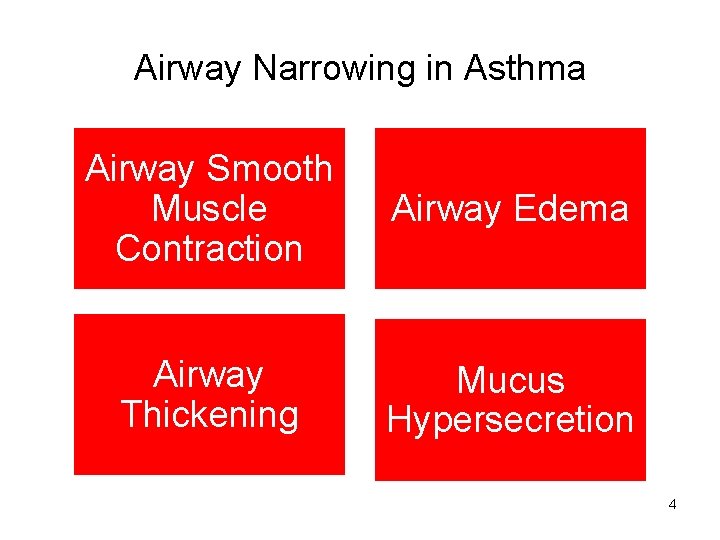
Airway Narrowing in Asthma Airway Smooth Muscle Contraction Airway Edema Airway Thickening Mucus Hypersecretion 4
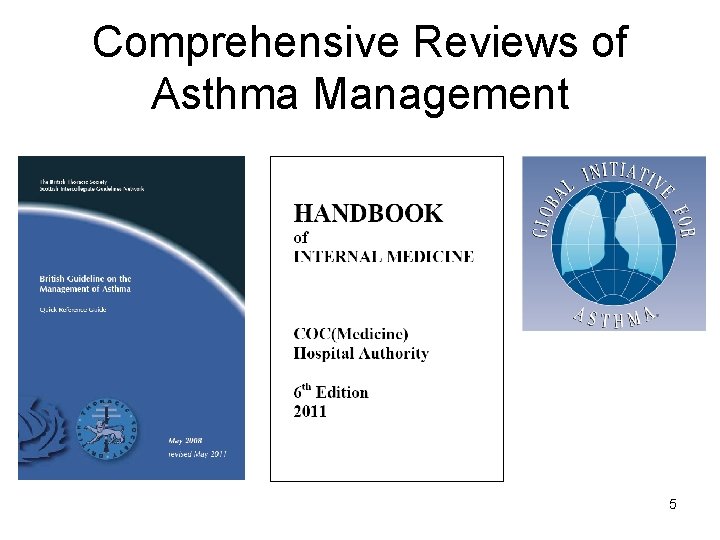
Comprehensive Reviews of Asthma Management 5
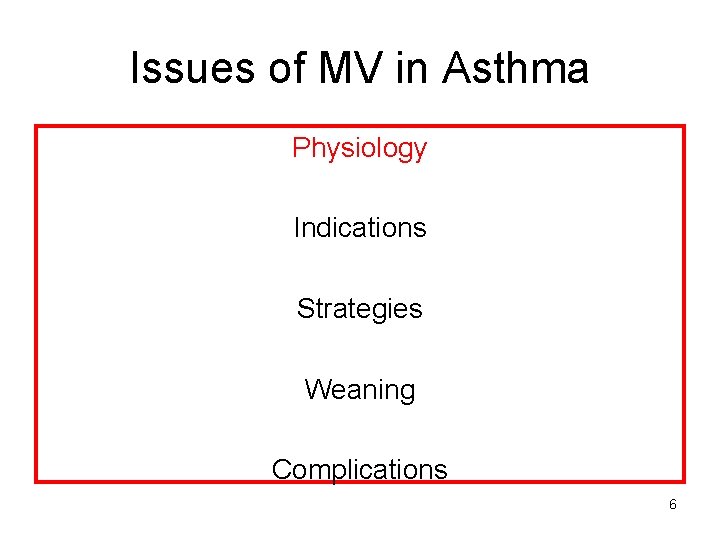
Issues of MV in Asthma Physiology Indications Strategies Weaning Complications 6
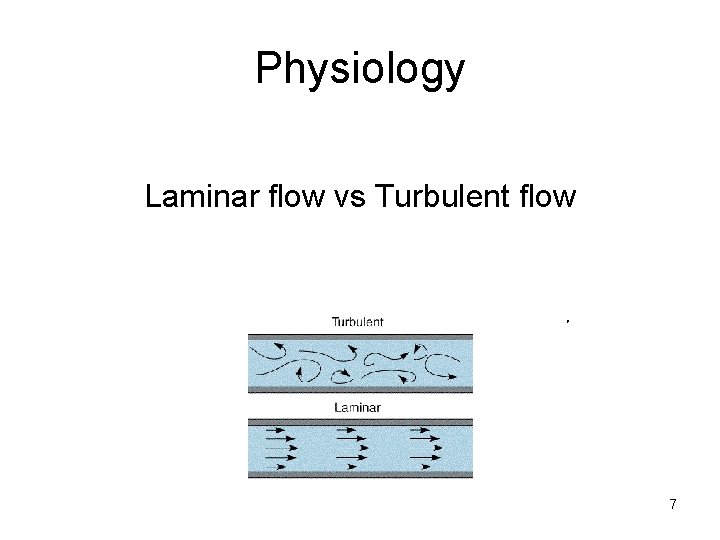
Physiology Laminar flow vs Turbulent flow . 7
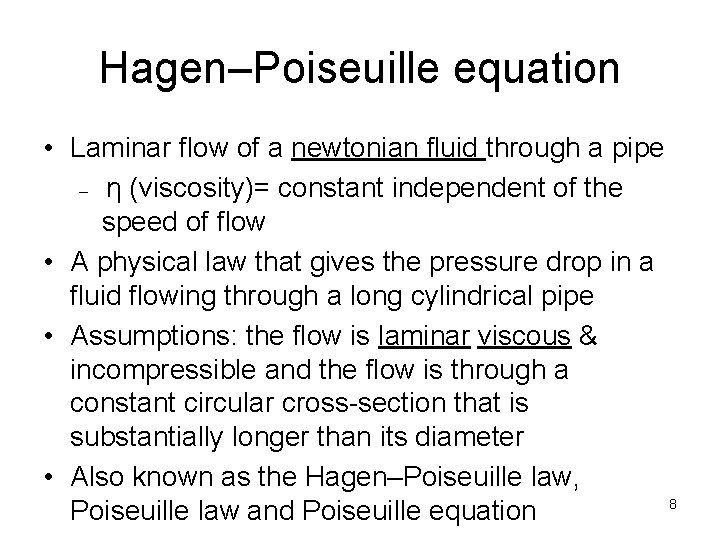
Hagen–Poiseuille equation • Laminar flow of a newtonian fluid through a pipe – η (viscosity)= constant independent of the speed of flow • A physical law that gives the pressure drop in a fluid flowing through a long cylindrical pipe • Assumptions: the flow is laminar viscous & incompressible and the flow is through a constant circular cross-section that is substantially longer than its diameter • Also known as the Hagen–Poiseuille law, 8 Poiseuille law and Poiseuille equation
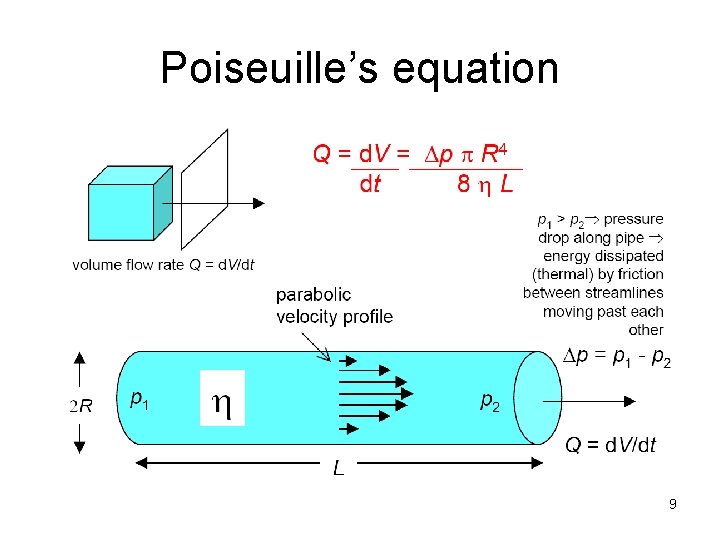
Poiseuille’s equation 9
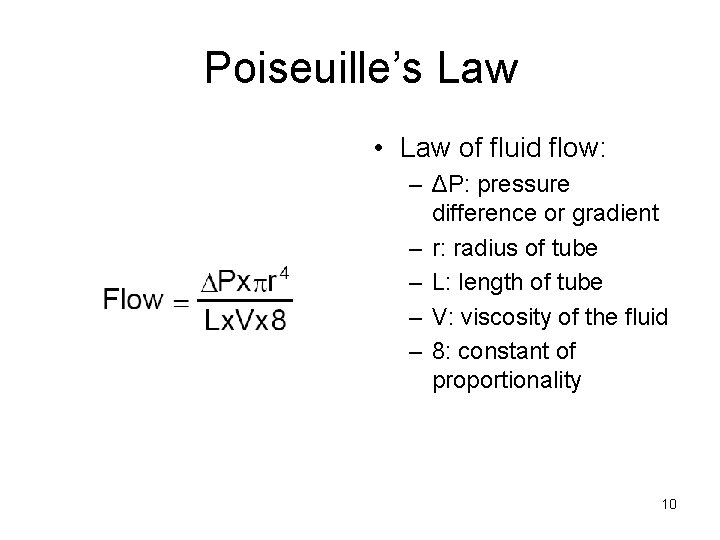
Poiseuille’s Law • Law of fluid flow: – ΔP: pressure difference or gradient – r: radius of tube – L: length of tube – V: viscosity of the fluid – 8: constant of proportionality 10
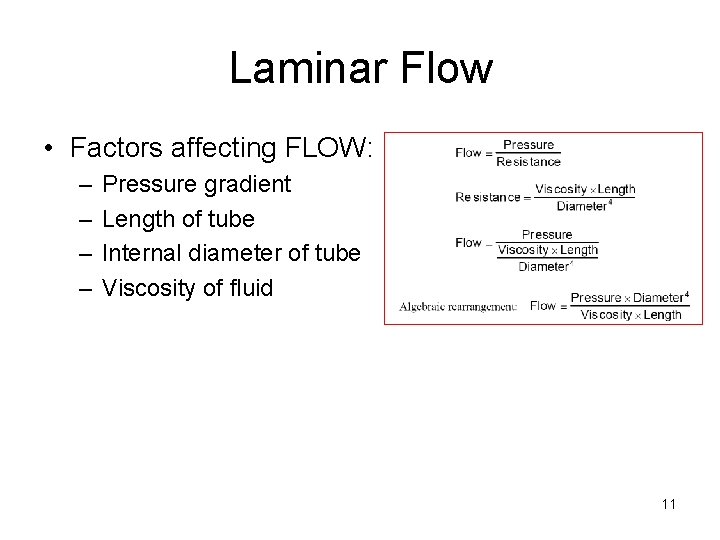
Laminar Flow • Factors affecting FLOW: – – Pressure gradient Length of tube Internal diameter of tube Viscosity of fluid 11
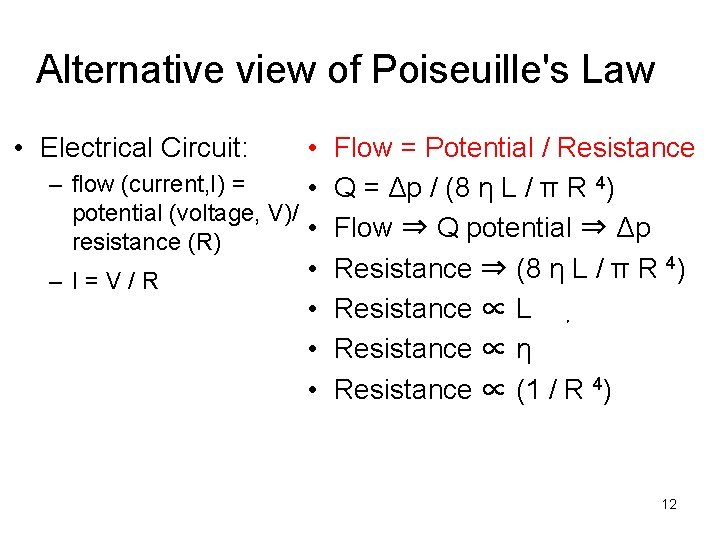
Alternative view of Poiseuille's Law • Electrical Circuit: • – flow (current, I) = • potential (voltage, V)/ • resistance (R) • – I=V/R • • • Flow = Potential / Resistance Q = Δp / (8 η L / π R 4) Flow ⇒ Q potential ⇒ Δp Resistance ⇒ (8 η L / π R 4) Resistance ∝ L . Resistance ∝ η Resistance ∝ (1 / R 4) 12
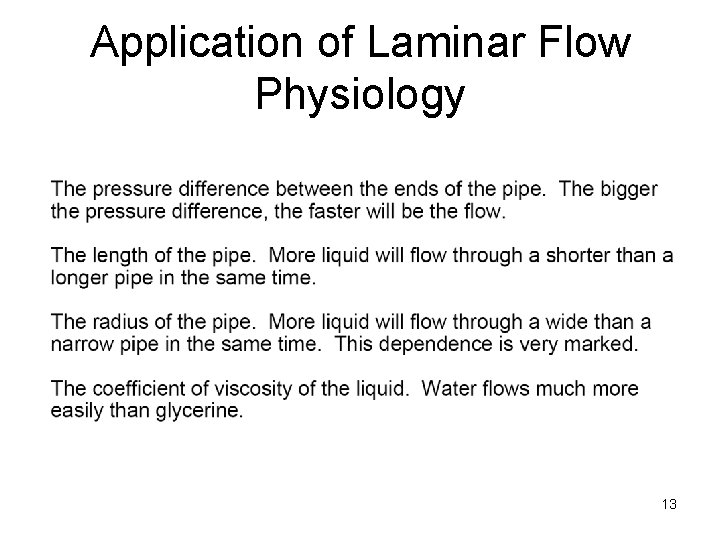
Application of Laminar Flow Physiology 13
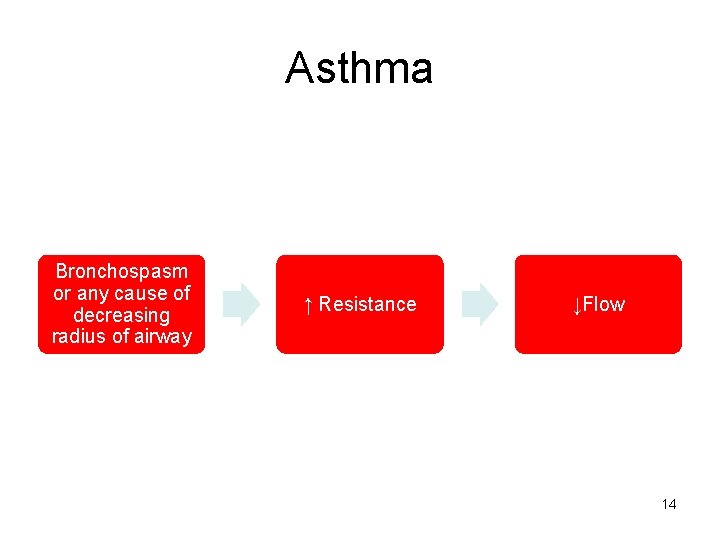
Asthma Bronchospasm or any cause of decreasing radius of airway ↑ Resistance ↓Flow 14
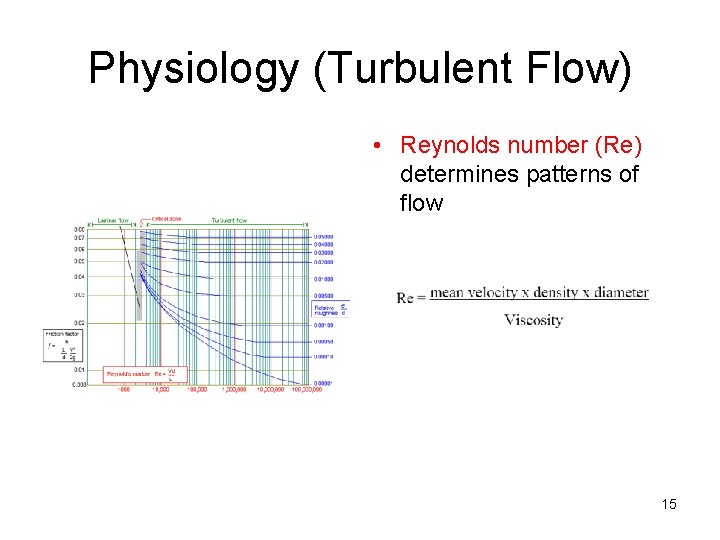
Physiology (Turbulent Flow) • Reynolds number (Re) determines patterns of flow 15
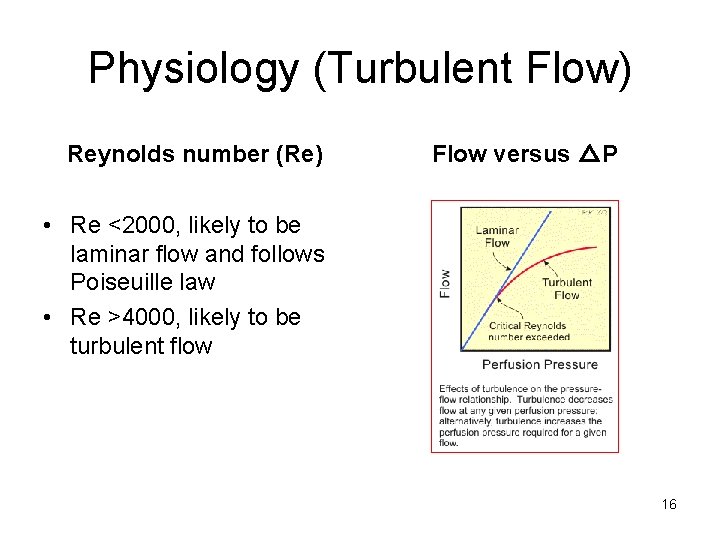
Physiology (Turbulent Flow) Reynolds number (Re) Flow versus △P • Re <2000, likely to be laminar flow and follows Poiseuille law • Re >4000, likely to be turbulent flow 16
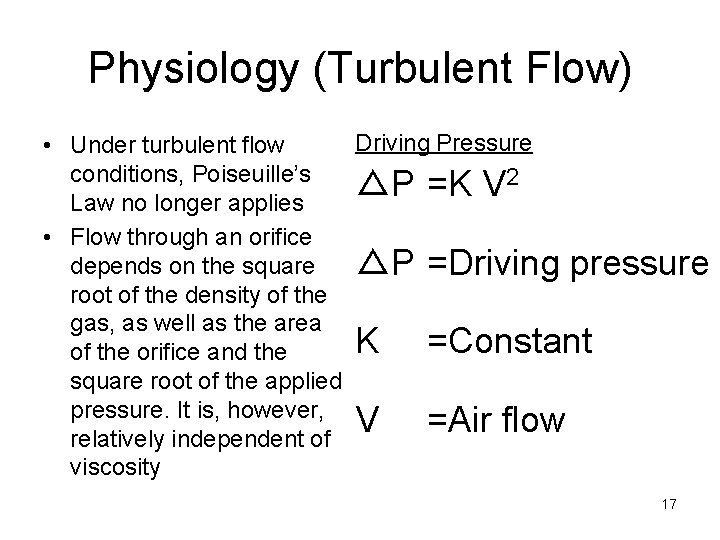
Physiology (Turbulent Flow) Driving Pressure • Under turbulent flow conditions, Poiseuille’s 2 △P =K V Law no longer applies • Flow through an orifice depends on the square △P =Driving pressure root of the density of the gas, as well as the area K =Constant of the orifice and the square root of the applied pressure. It is, however, V =Air flow relatively independent of viscosity 17
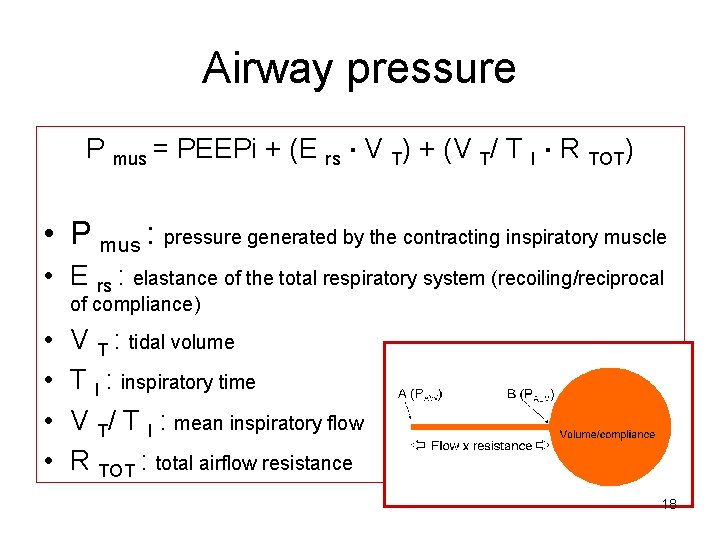
Airway pressure P mus = PEEPi + (E rs. V T) + (V T/ T I. R TOT) • P mus : pressure generated by the contracting inspiratory muscle • E rs : elastance of the total respiratory system (recoiling/reciprocal of compliance) • • V T : tidal volume T I : inspiratory time V T/ T I : mean inspiratory flow R TOT : total airflow resistance 18
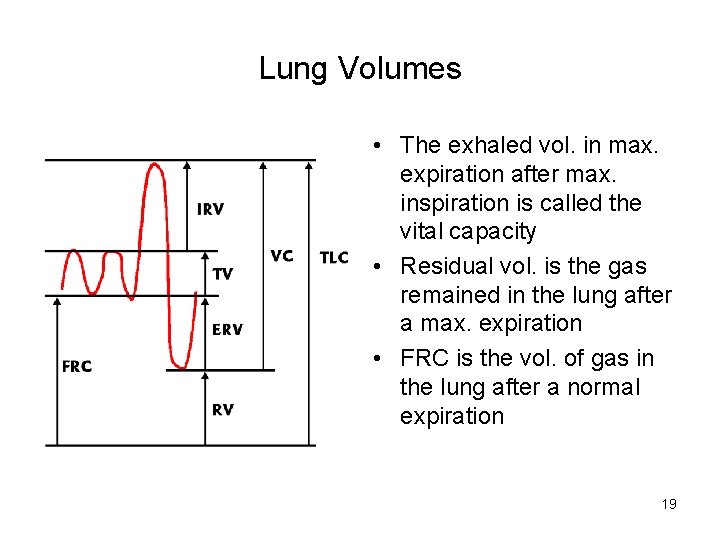
Lung Volumes • The exhaled vol. in max. expiration after max. inspiration is called the vital capacity • Residual vol. is the gas remained in the lung after a max. expiration • FRC is the vol. of gas in the lung after a normal expiration 19
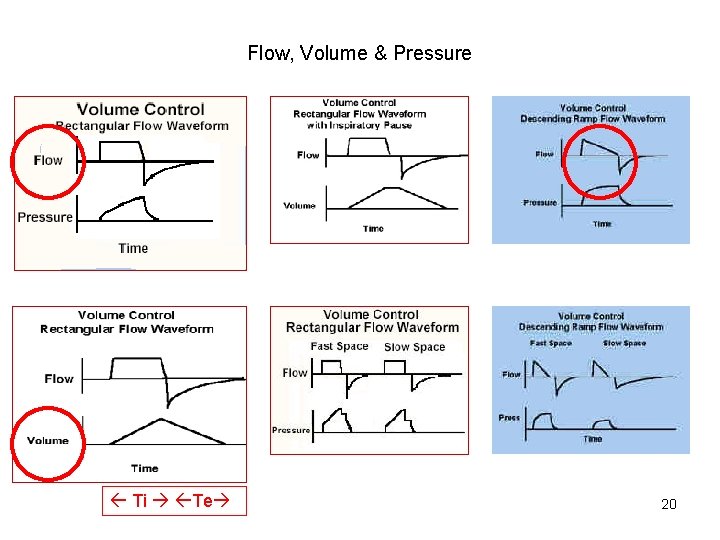
Flow, Volume & Pressure Ti Te 20
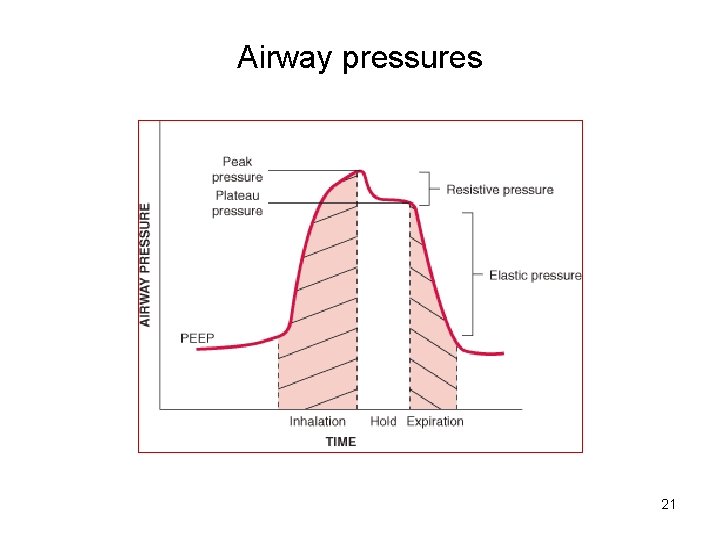
Airway pressures 21
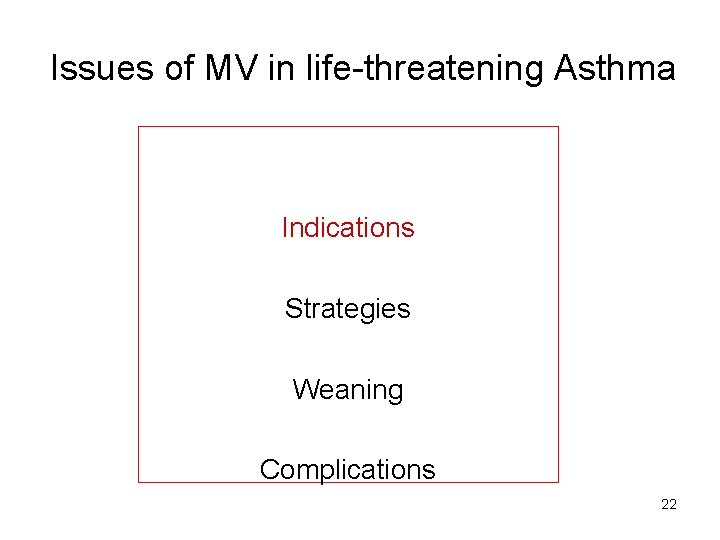
Issues of MV in life-threatening Asthma Indications Strategies Weaning Complications 22
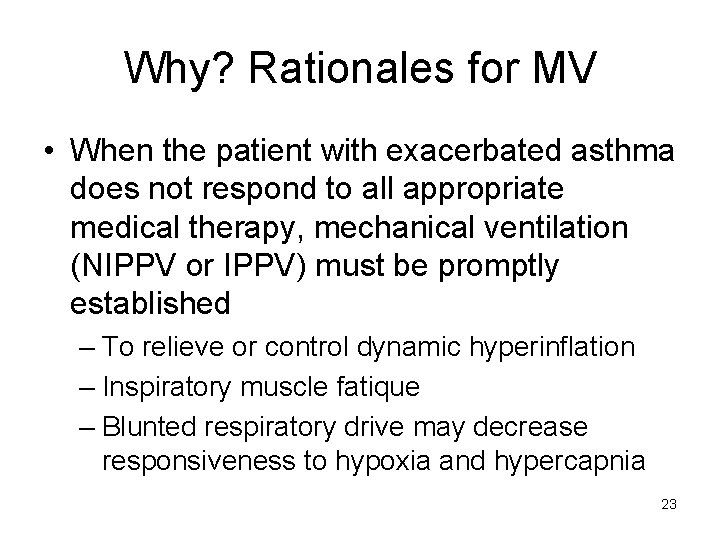
Why? Rationales for MV • When the patient with exacerbated asthma does not respond to all appropriate medical therapy, mechanical ventilation (NIPPV or IPPV) must be promptly established – To relieve or control dynamic hyperinflation – Inspiratory muscle fatique – Blunted respiratory drive may decrease responsiveness to hypoxia and hypercapnia 23
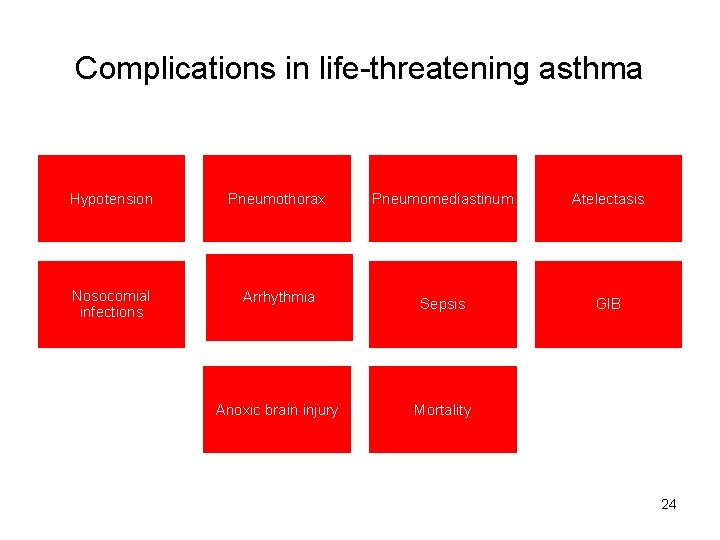
Complications in life-threatening asthma Hypotension Pneumothorax Pneumomediastinum Atelectasis Nosocomial infections Arrhythmia Sepsis GIB Anoxic brain injury Mortality 24
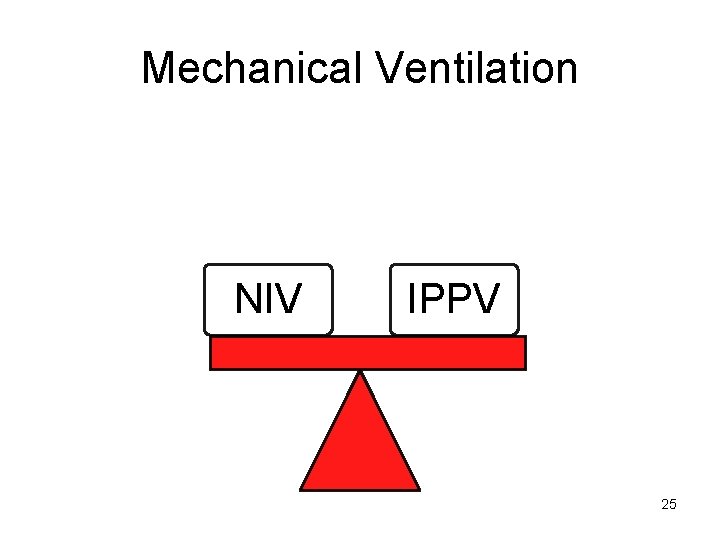
Mechanical Ventilation NIV IPPV 25
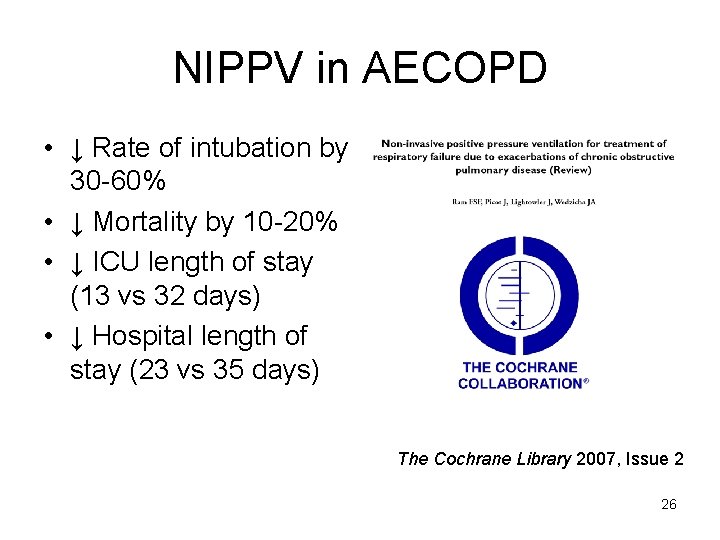
NIPPV in AECOPD • ↓ Rate of intubation by 30 -60% • ↓ Mortality by 10 -20% • ↓ ICU length of stay (13 vs 32 days) • ↓ Hospital length of stay (23 vs 35 days) The Cochrane Library 2007, Issue 2 26
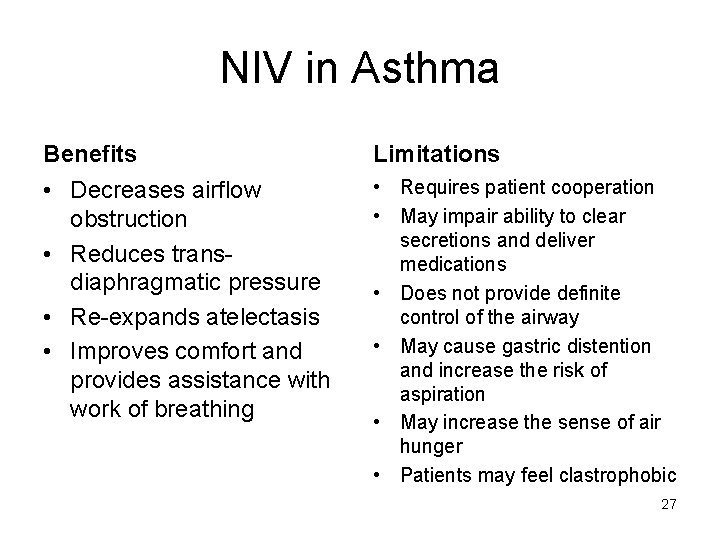
NIV in Asthma Benefits Limitations • Decreases airflow obstruction • Reduces transdiaphragmatic pressure • Re-expands atelectasis • Improves comfort and provides assistance with work of breathing • Requires patient cooperation • May impair ability to clear secretions and deliver medications • Does not provide definite control of the airway • May cause gastric distention and increase the risk of aspiration • May increase the sense of air hunger • Patients may feel clastrophobic 27
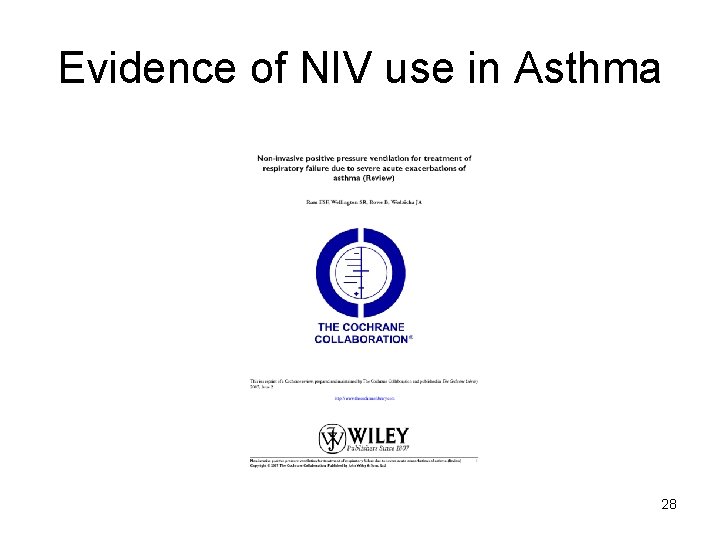
Evidence of NIV use in Asthma 28
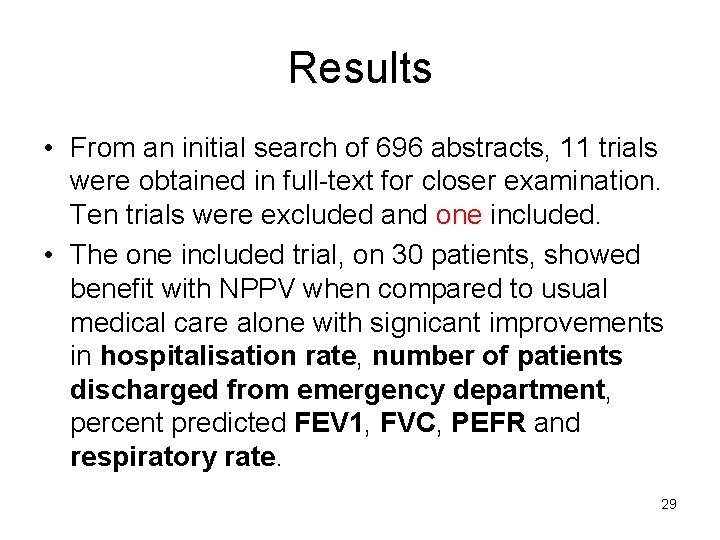
Results • From an initial search of 696 abstracts, 11 trials were obtained in full-text for closer examination. Ten trials were excluded and one included. • The one included trial, on 30 patients, showed benefit with NPPV when compared to usual medical care alone with signicant improvements in hospitalisation rate, number of patients discharged from emergency department, percent predicted FEV 1, FVC, PEFR and respiratory rate. 29
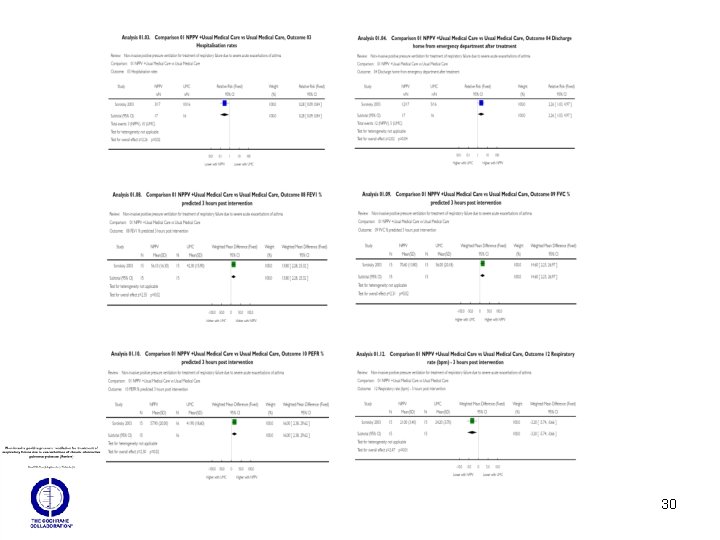
30
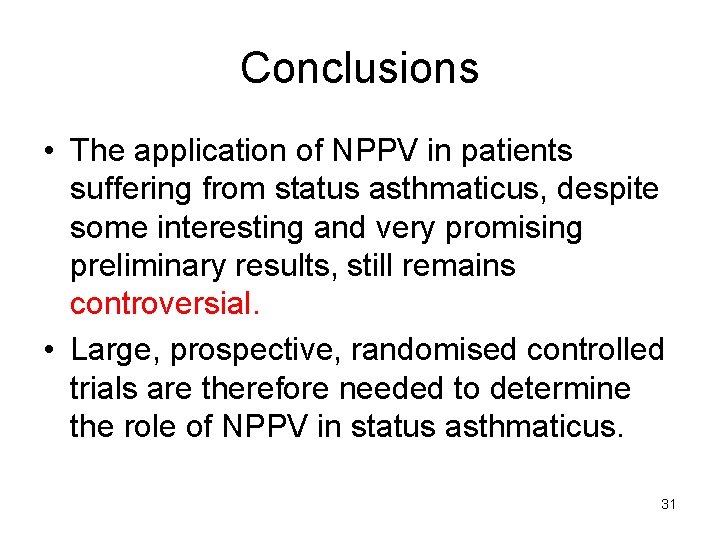
Conclusions • The application of NPPV in patients suffering from status asthmaticus, despite some interesting and very promising preliminary results, still remains controversial. • Large, prospective, randomised controlled trials are therefore needed to determine the role of NPPV in status asthmaticus. 31
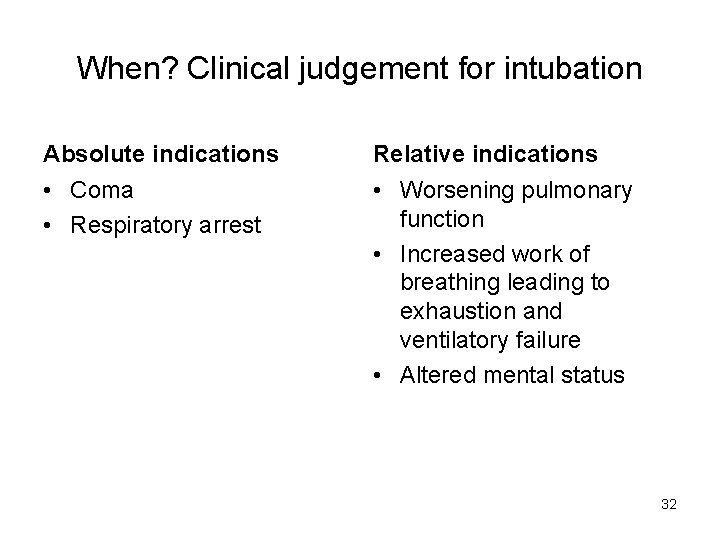
When? Clinical judgement for intubation Absolute indications Relative indications • Coma • Respiratory arrest • Worsening pulmonary function • Increased work of breathing leading to exhaustion and ventilatory failure • Altered mental status 32
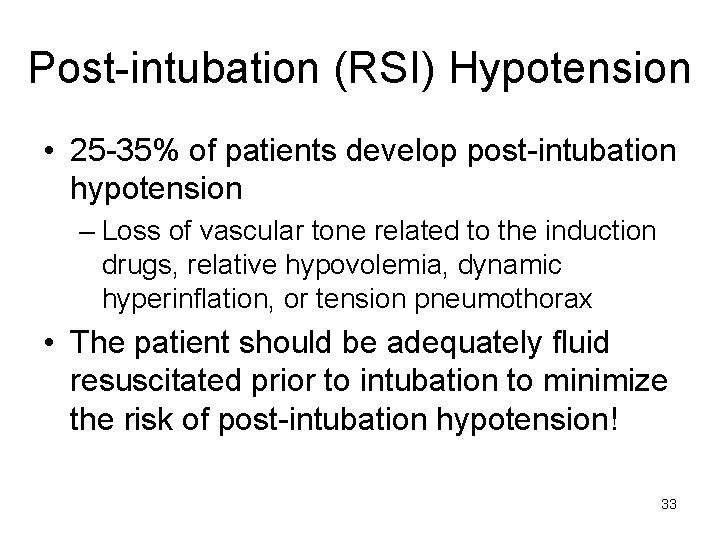
Post-intubation (RSI) Hypotension • 25 -35% of patients develop post-intubation hypotension – Loss of vascular tone related to the induction drugs, relative hypovolemia, dynamic hyperinflation, or tension pneumothorax • The patient should be adequately fluid resuscitated prior to intubation to minimize the risk of post-intubation hypotension! 33
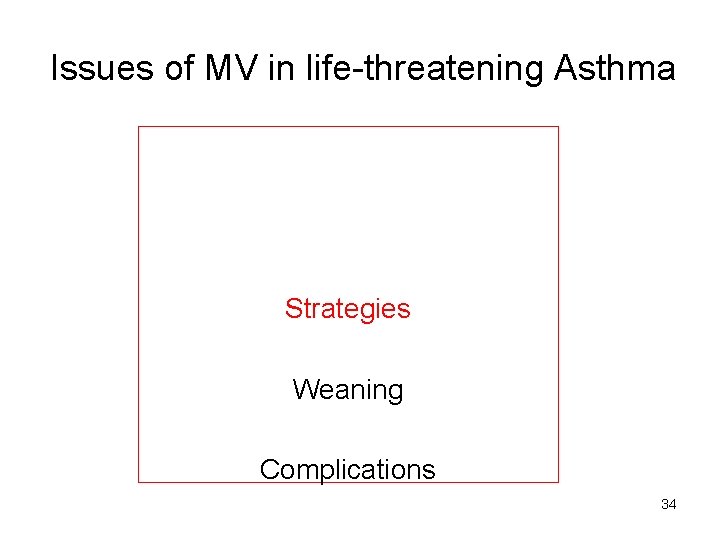
Issues of MV in life-threatening Asthma Strategies Weaning Complications 34
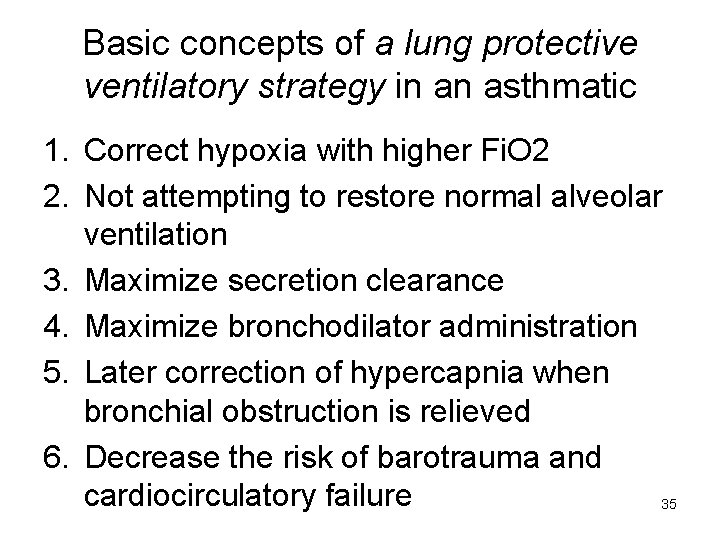
Basic concepts of a lung protective ventilatory strategy in an asthmatic 1. Correct hypoxia with higher Fi. O 2 2. Not attempting to restore normal alveolar ventilation 3. Maximize secretion clearance 4. Maximize bronchodilator administration 5. Later correction of hypercapnia when bronchial obstruction is relieved 6. Decrease the risk of barotrauma and cardiocirculatory failure 35
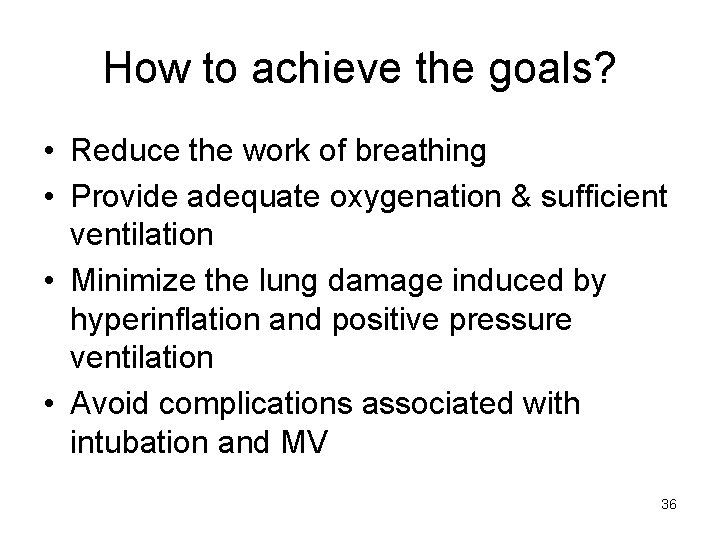
How to achieve the goals? • Reduce the work of breathing • Provide adequate oxygenation & sufficient ventilation • Minimize the lung damage induced by hyperinflation and positive pressure ventilation • Avoid complications associated with intubation and MV 36
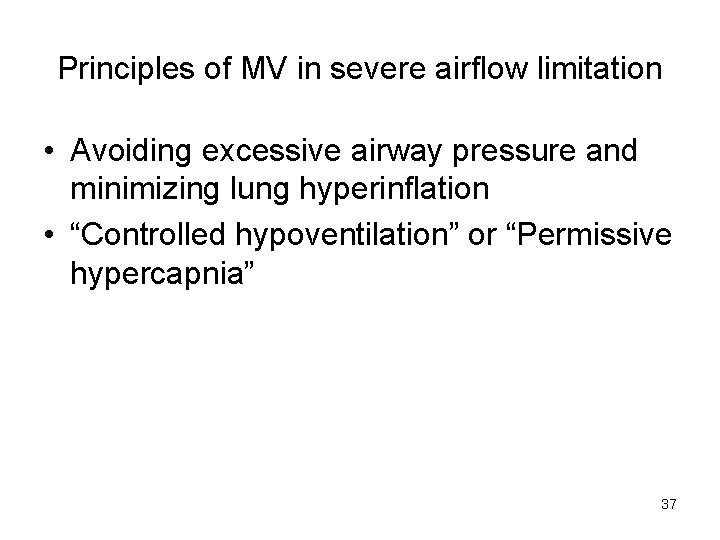
Principles of MV in severe airflow limitation • Avoiding excessive airway pressure and minimizing lung hyperinflation • “Controlled hypoventilation” or “Permissive hypercapnia” 37
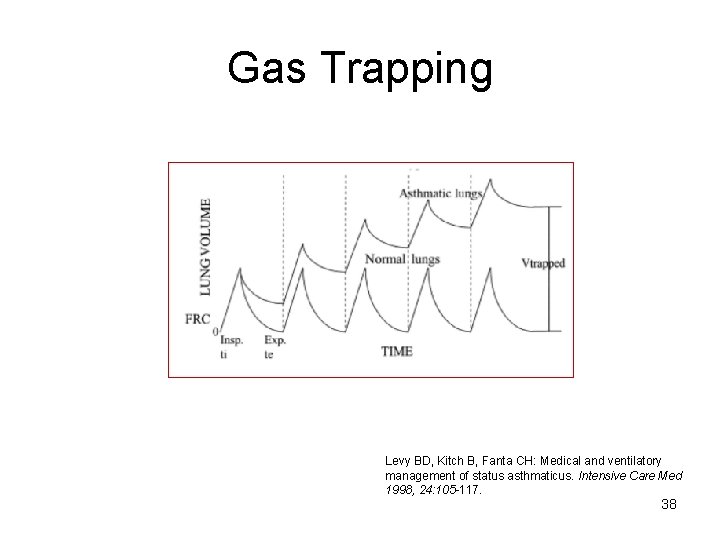
Gas Trapping Levy BD, Kitch B, Fanta CH: Medical and ventilatory management of status asthmaticus. Intensive Care Med 1998, 24: 105 -117. 38
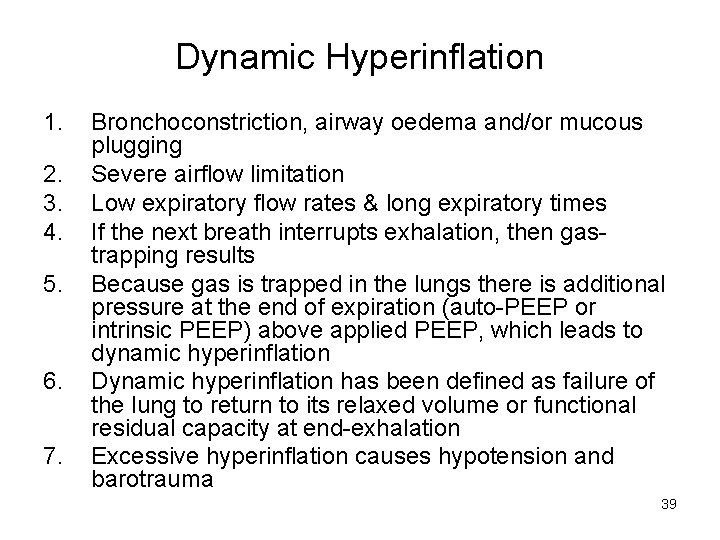
Dynamic Hyperinflation 1. 2. 3. 4. 5. 6. 7. Bronchoconstriction, airway oedema and/or mucous plugging Severe airflow limitation Low expiratory flow rates & long expiratory times If the next breath interrupts exhalation, then gastrapping results Because gas is trapped in the lungs there is additional pressure at the end of expiration (auto-PEEP or intrinsic PEEP) above applied PEEP, which leads to dynamic hyperinflation Dynamic hyperinflation has been defined as failure of the lung to return to its relaxed volume or functional residual capacity at end-exhalation Excessive hyperinflation causes hypotension and barotrauma 39
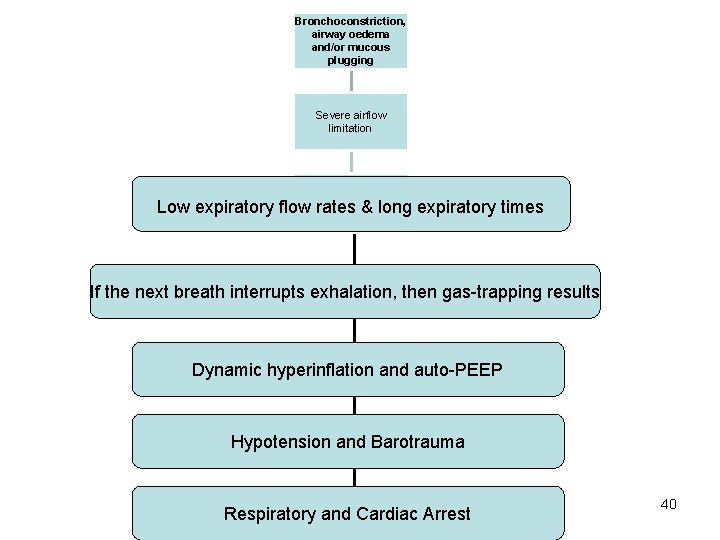
Bronchoconstriction, airway oedema and/or mucous plugging Severe airflow limitation Low expiratory flow rates & long expiratory times If the next breath interrupts exhalation, then gas-trapping results Dynamic hyperinflation and auto-PEEP Hypotension and Barotrauma Respiratory and Cardiac Arrest 40
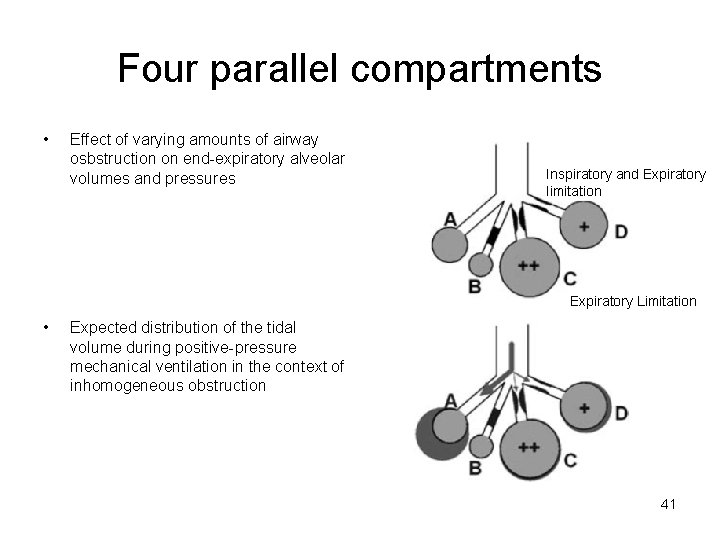
Four parallel compartments • Effect of varying amounts of airway osbstruction on end-expiratory alveolar volumes and pressures Inspiratory and Expiratory limitation Expiratory Limitation • Expected distribution of the tidal volume during positive-pressure mechanical ventilation in the context of inhomogeneous obstruction 41
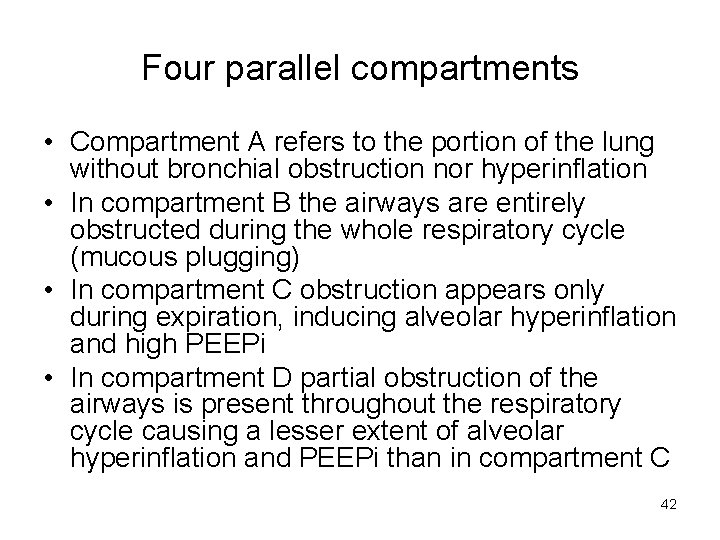
Four parallel compartments • Compartment A refers to the portion of the lung without bronchial obstruction nor hyperinflation • In compartment B the airways are entirely obstructed during the whole respiratory cycle (mucous plugging) • In compartment C obstruction appears only during expiration, inducing alveolar hyperinflation and high PEEPi • In compartment D partial obstruction of the airways is present throughout the respiratory cycle causing a lesser extent of alveolar hyperinflation and PEEPi than in compartment C 42
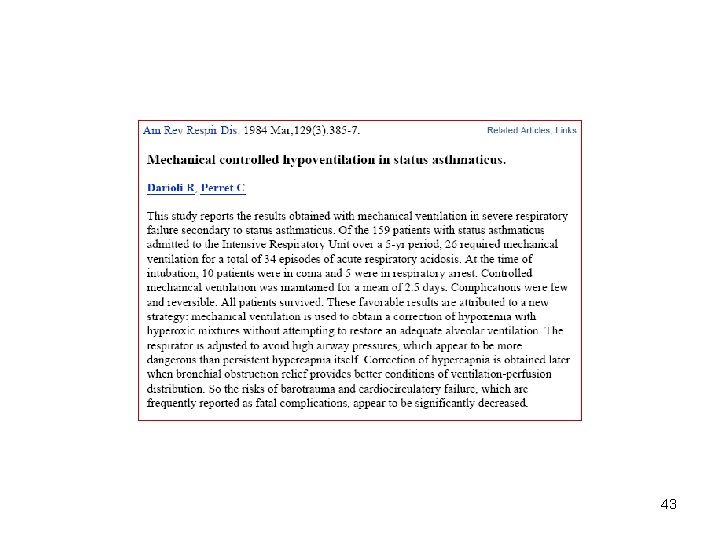
43
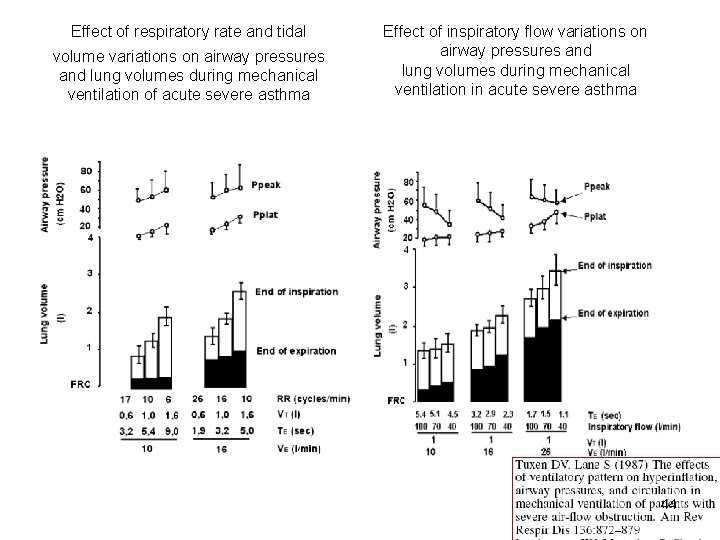
Effect of respiratory rate and tidal volume variations on airway pressures and lung volumes during mechanical ventilation of acute severe asthma Effect of inspiratory flow variations on airway pressures and lung volumes during mechanical ventilation in acute severe asthma 44
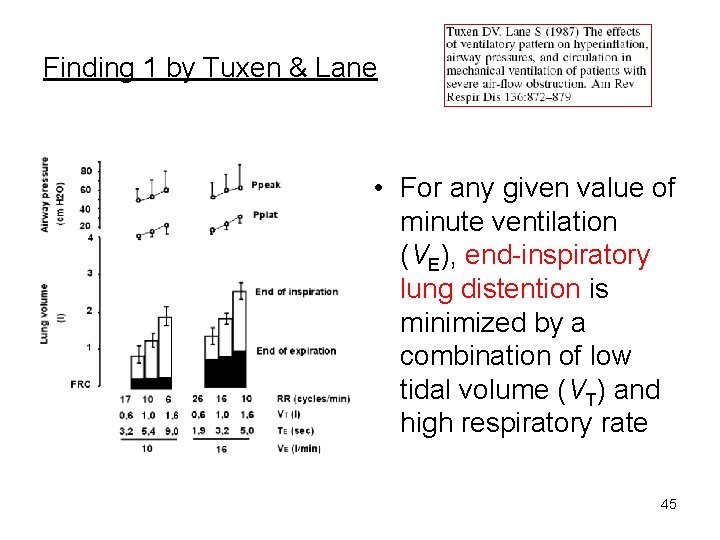
Finding 1 by Tuxen & Lane • For any given value of minute ventilation (VE), end-inspiratory lung distention is minimized by a combination of low tidal volume (VT) and high respiratory rate 45
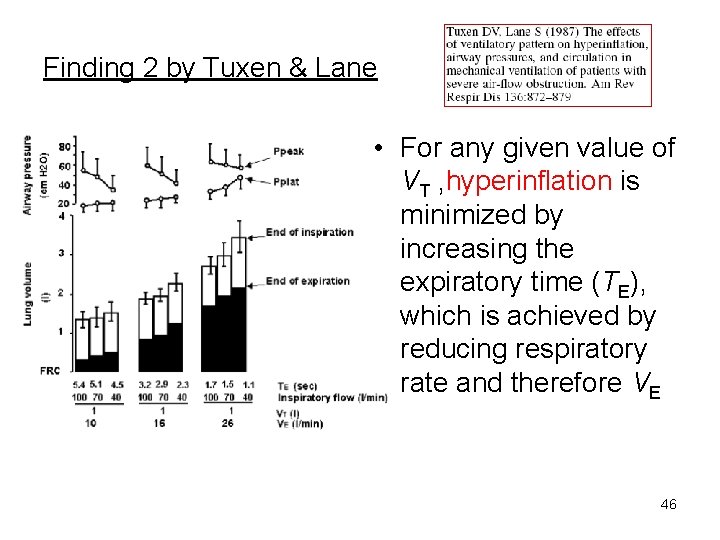
Finding 2 by Tuxen & Lane • For any given value of VT , hyperinflation is minimized by increasing the expiratory time (TE), which is achieved by reducing respiratory rate and therefore VE 46
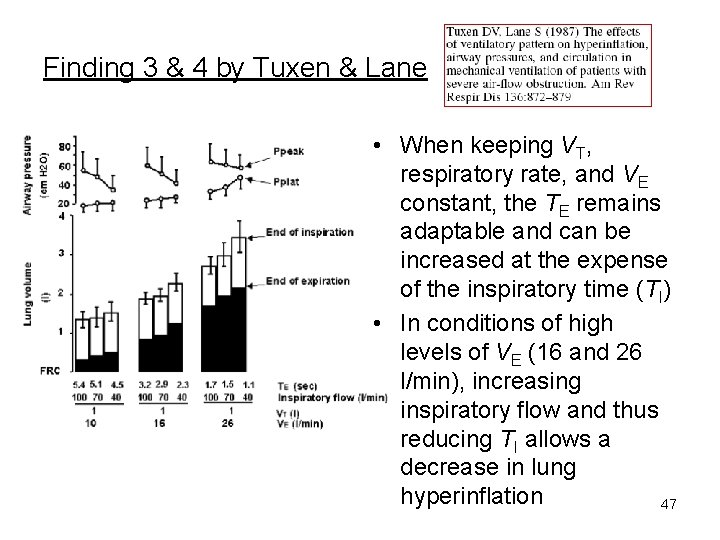
Finding 3 & 4 by Tuxen & Lane • When keeping VT, respiratory rate, and VE constant, the TE remains adaptable and can be increased at the expense of the inspiratory time (TI) • In conditions of high levels of VE (16 and 26 l/min), increasing inspiratory flow and thus reducing TI allows a decrease in lung hyperinflation 47
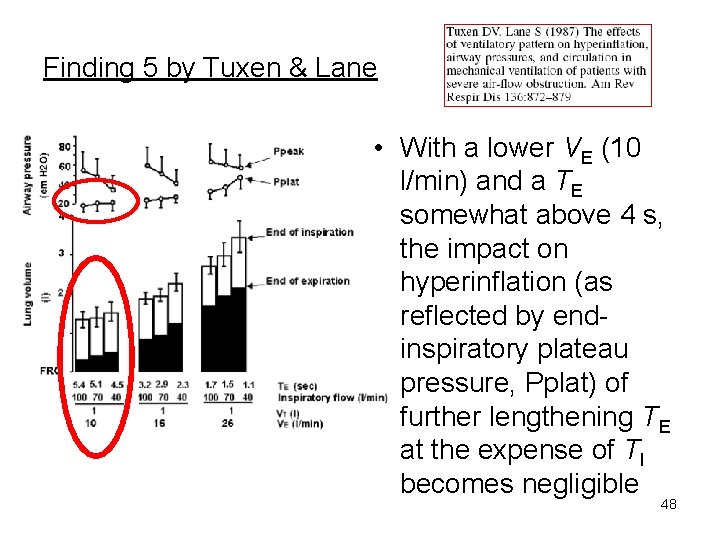
Finding 5 by Tuxen & Lane • With a lower VE (10 l/min) and a TE somewhat above 4 s, the impact on hyperinflation (as reflected by endinspiratory plateau pressure, Pplat) of further lengthening TE at the expense of TI becomes negligible 48
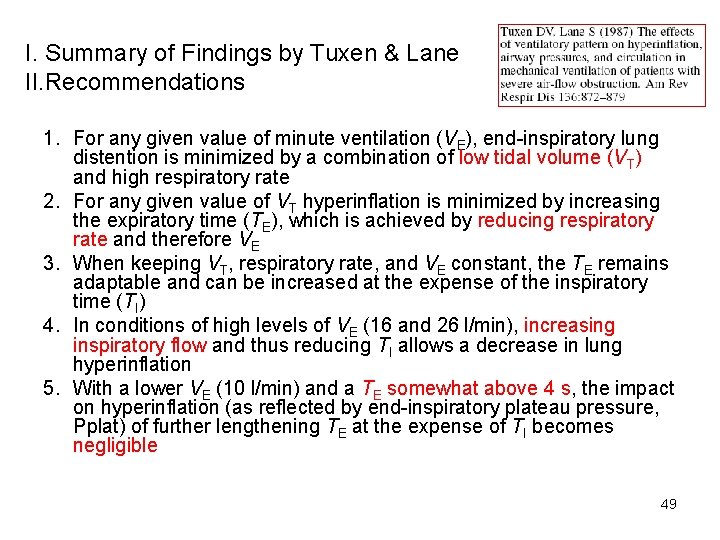
I. Summary of Findings by Tuxen & Lane II. Recommendations 1. For any given value of minute ventilation (VE), end-inspiratory lung distention is minimized by a combination of low tidal volume (VT) and high respiratory rate 2. For any given value of VT hyperinflation is minimized by increasing the expiratory time (TE), which is achieved by reducing respiratory rate and therefore VE 3. When keeping VT, respiratory rate, and VE constant, the TE remains adaptable and can be increased at the expense of the inspiratory time (TI) 4. In conditions of high levels of VE (16 and 26 l/min), increasing inspiratory flow and thus reducing TI allows a decrease in lung hyperinflation 5. With a lower VE (10 l/min) and a TE somewhat above 4 s, the impact on hyperinflation (as reflected by end-inspiratory plateau pressure, Pplat) of further lengthening TE at the expense of TI becomes negligible 49
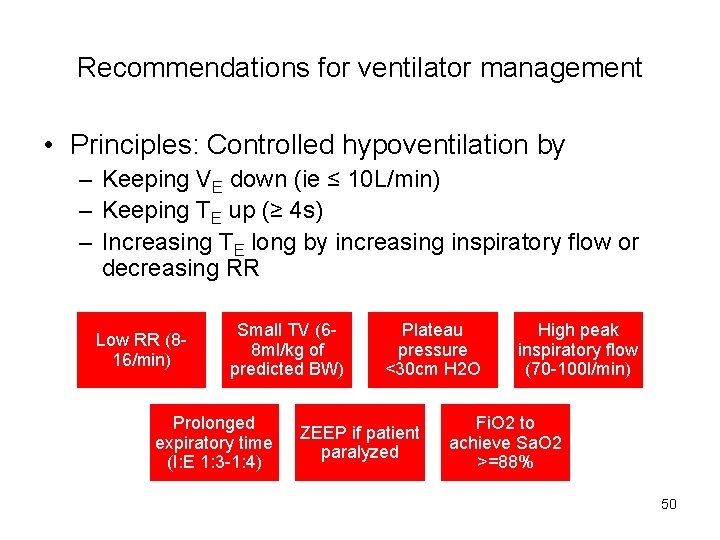
Recommendations for ventilator management • Principles: Controlled hypoventilation by – Keeping VE down (ie ≤ 10 L/min) – Keeping TE up (≥ 4 s) – Increasing TE long by increasing inspiratory flow or decreasing RR Low RR (816/min) Small TV (68 ml/kg of predicted BW) Prolonged expiratory time (I: E 1: 3 -1: 4) Plateau pressure <30 cm H 2 O ZEEP if patient paralyzed High peak inspiratory flow (70 -100 l/min) Fi. O 2 to achieve Sa. O 2 >=88% 50
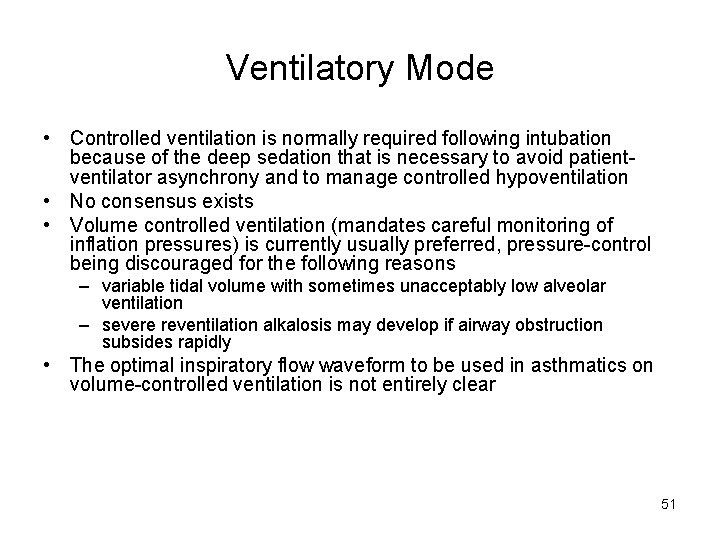
Ventilatory Mode • Controlled ventilation is normally required following intubation because of the deep sedation that is necessary to avoid patientventilator asynchrony and to manage controlled hypoventilation • No consensus exists • Volume controlled ventilation (mandates careful monitoring of inflation pressures) is currently usually preferred, pressure-control being discouraged for the following reasons – variable tidal volume with sometimes unacceptably low alveolar ventilation – severe reventilation alkalosis may develop if airway obstruction subsides rapidly • The optimal inspiratory flow waveform to be used in asthmatics on volume-controlled ventilation is not entirely clear 51
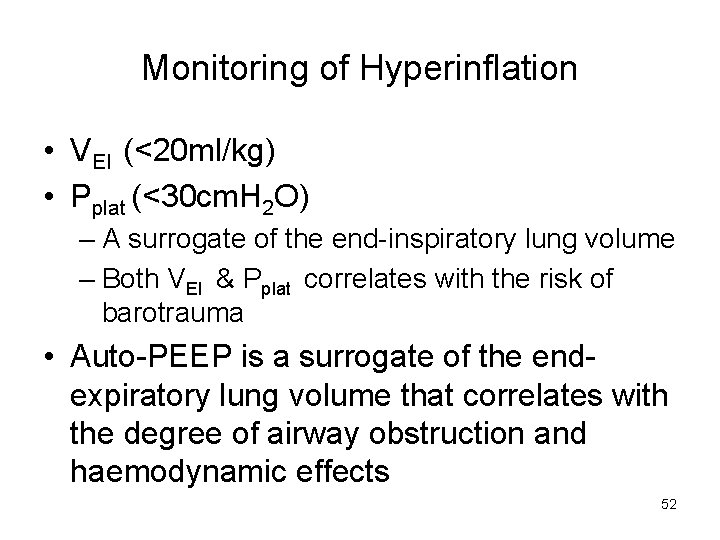
Monitoring of Hyperinflation • VEI (<20 ml/kg) • Pplat (<30 cm. H 2 O) – A surrogate of the end-inspiratory lung volume – Both VEI & Pplat correlates with the risk of barotrauma • Auto-PEEP is a surrogate of the endexpiratory lung volume that correlates with the degree of airway obstruction and haemodynamic effects 52
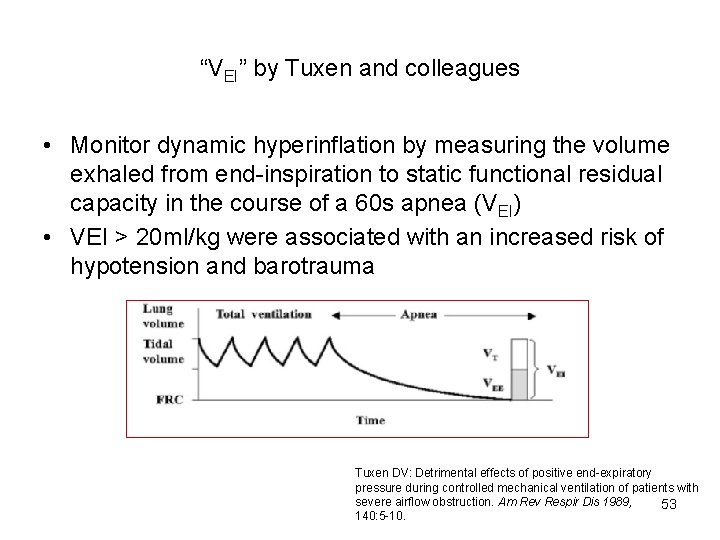
“VEI” by Tuxen and colleagues • Monitor dynamic hyperinflation by measuring the volume exhaled from end-inspiration to static functional residual capacity in the course of a 60 s apnea (VEI) • VEI > 20 ml/kg were associated with an increased risk of hypotension and barotrauma Tuxen DV: Detrimental effects of positive end-expiratory pressure during controlled mechanical ventilation of patients with severe airflow obstruction. Am Rev Respir Dis 1989, 53 140: 5 -10.
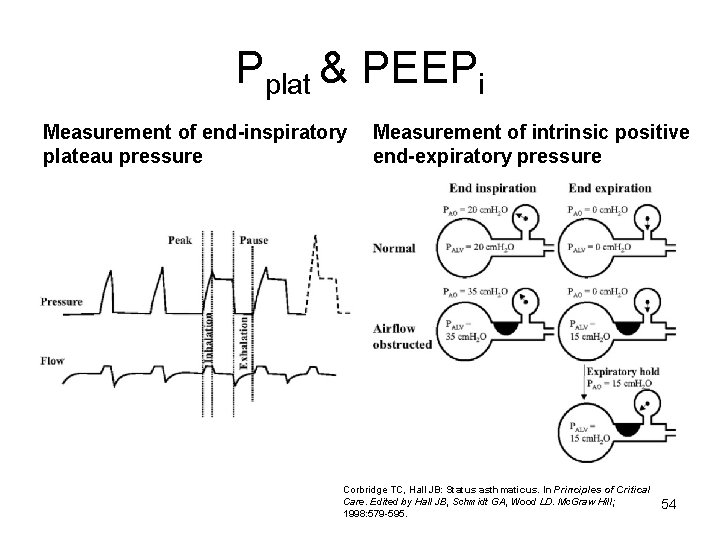
Pplat & PEEPi Measurement of end-inspiratory plateau pressure Measurement of intrinsic positive end-expiratory pressure Corbridge TC, Hall JB: Status asthmaticus. In Principles of Critical Care. Edited by Hall JB, Schmidt GA, Wood LD. Mc. Graw Hill; 1998: 579 -595. 54
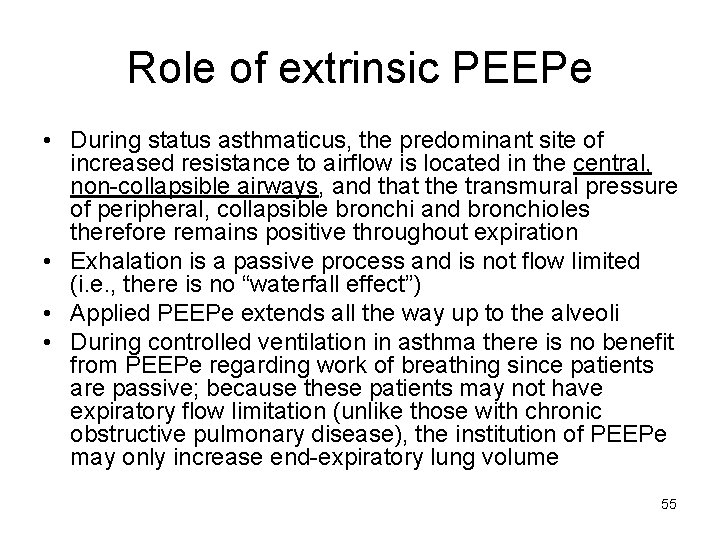
Role of extrinsic PEEPe • During status asthmaticus, the predominant site of increased resistance to airflow is located in the central, non-collapsible airways, and that the transmural pressure of peripheral, collapsible bronchi and bronchioles therefore remains positive throughout expiration • Exhalation is a passive process and is not flow limited (i. e. , there is no “waterfall effect”) • Applied PEEPe extends all the way up to the alveoli • During controlled ventilation in asthma there is no benefit from PEEPe regarding work of breathing since patients are passive; because these patients may not have expiratory flow limitation (unlike those with chronic obstructive pulmonary disease), the institution of PEEPe may only increase end-expiratory lung volume 55
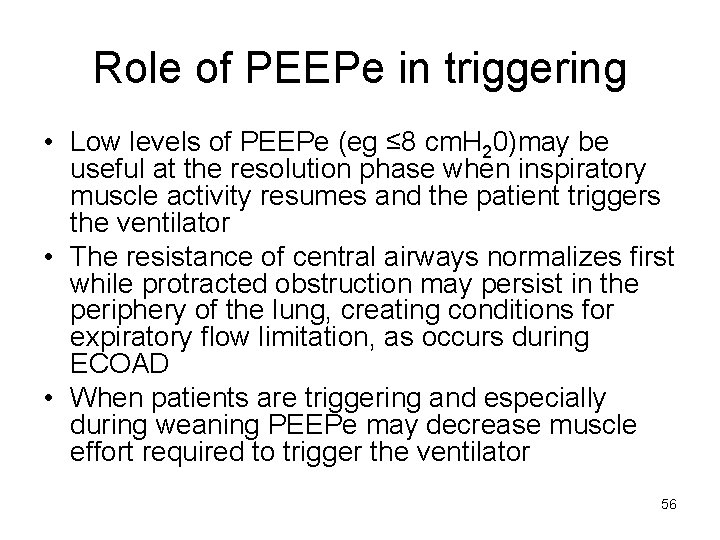
Role of PEEPe in triggering • Low levels of PEEPe (eg ≤ 8 cm. H 20)may be useful at the resolution phase when inspiratory muscle activity resumes and the patient triggers the ventilator • The resistance of central airways normalizes first while protracted obstruction may persist in the periphery of the lung, creating conditions for expiratory flow limitation, as occurs during ECOAD • When patients are triggering and especially during weaning PEEPe may decrease muscle effort required to trigger the ventilator 56
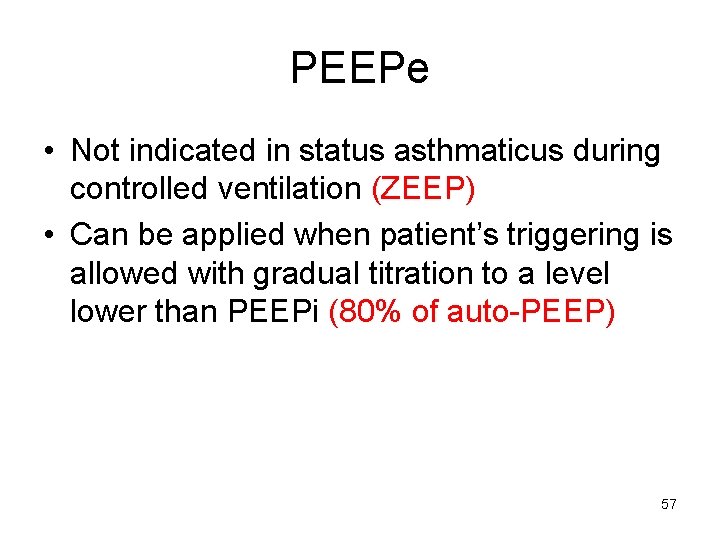
PEEPe • Not indicated in status asthmaticus during controlled ventilation (ZEEP) • Can be applied when patient’s triggering is allowed with gradual titration to a level lower than PEEPi (80% of auto-PEEP) 57
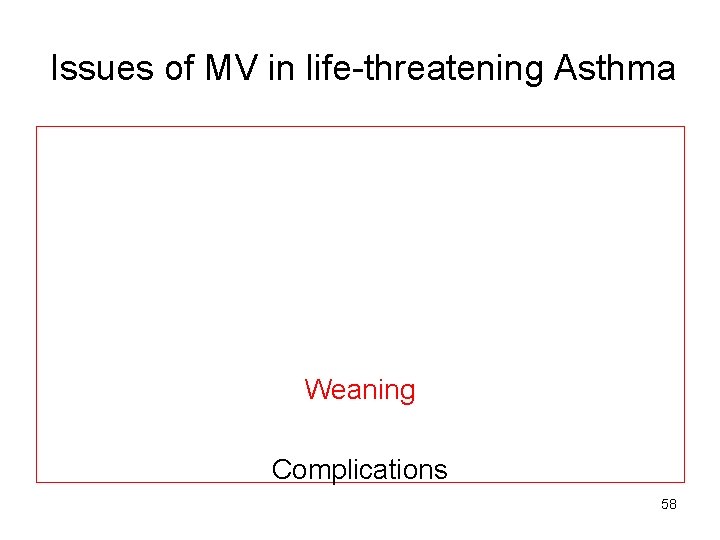
Issues of MV in life-threatening Asthma Weaning Complications 58
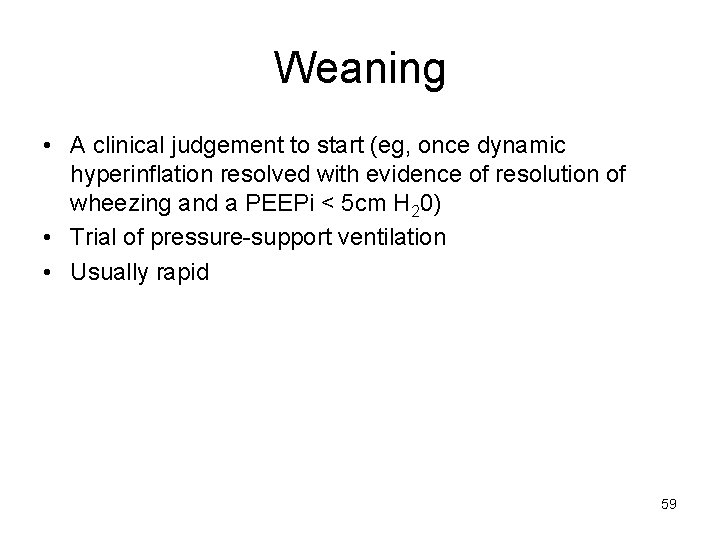
Weaning • A clinical judgement to start (eg, once dynamic hyperinflation resolved with evidence of resolution of wheezing and a PEEPi < 5 cm H 20) • Trial of pressure-support ventilation • Usually rapid 59
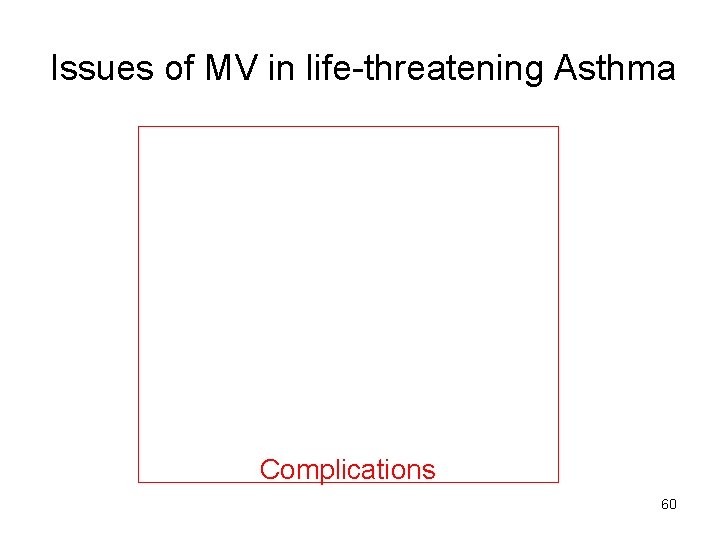
Issues of MV in life-threatening Asthma Complications 60
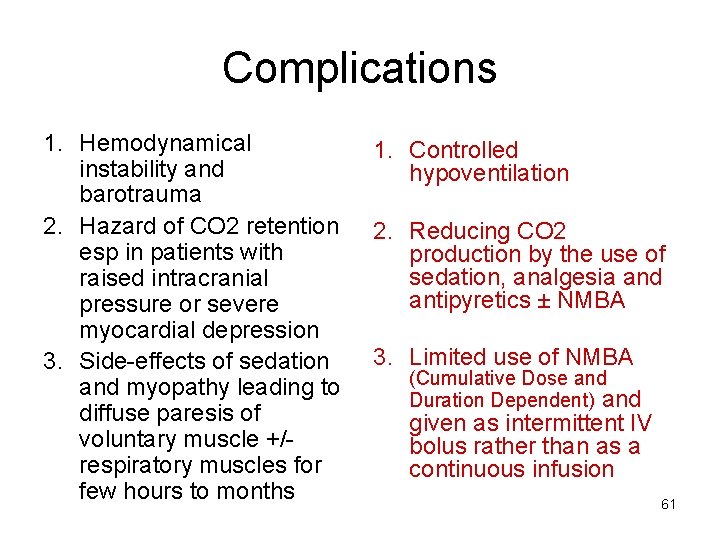
Complications 1. Hemodynamical instability and barotrauma 2. Hazard of CO 2 retention esp in patients with raised intracranial pressure or severe myocardial depression 3. Side-effects of sedation and myopathy leading to diffuse paresis of voluntary muscle +/respiratory muscles for few hours to months 1. Controlled hypoventilation 2. Reducing CO 2 production by the use of sedation, analgesia and antipyretics ± NMBA 3. Limited use of NMBA (Cumulative Dose and Duration Dependent) and given as intermittent IV bolus rather than as a continuous infusion 61
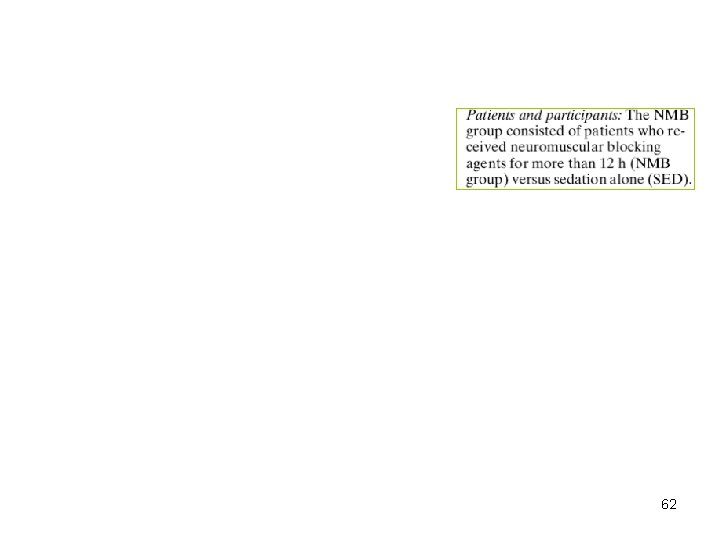
62
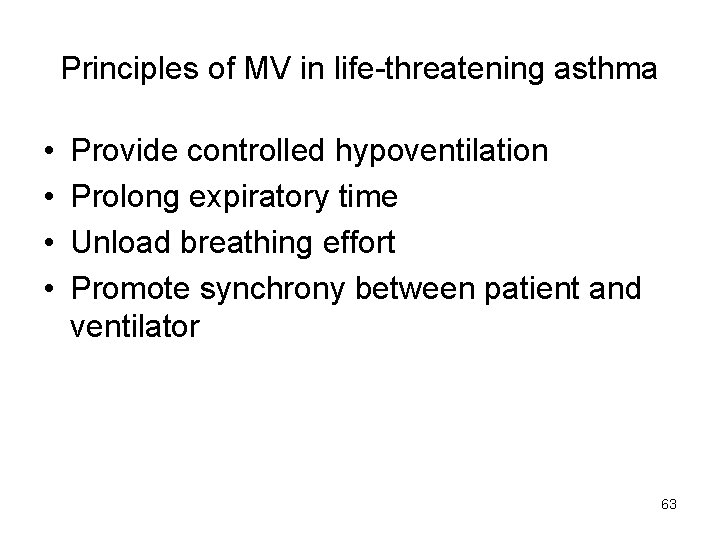
Principles of MV in life-threatening asthma • • Provide controlled hypoventilation Prolong expiratory time Unload breathing effort Promote synchrony between patient and ventilator 63
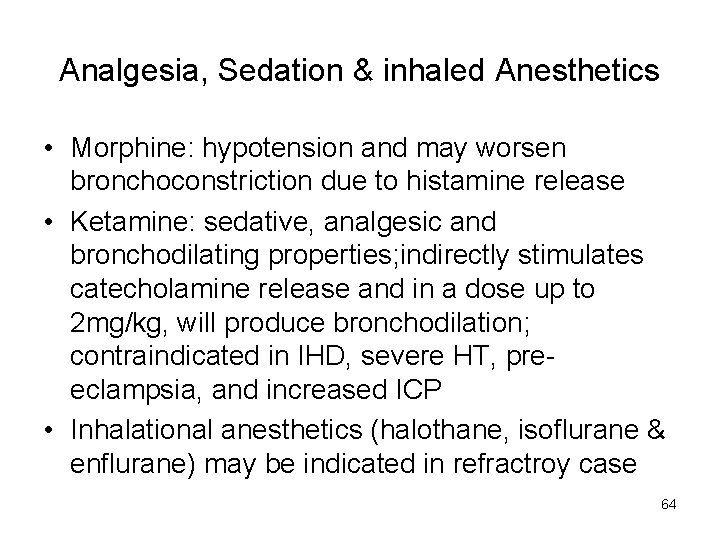
Analgesia, Sedation & inhaled Anesthetics • Morphine: hypotension and may worsen bronchoconstriction due to histamine release • Ketamine: sedative, analgesic and bronchodilating properties; indirectly stimulates catecholamine release and in a dose up to 2 mg/kg, will produce bronchodilation; contraindicated in IHD, severe HT, preeclampsia, and increased ICP • Inhalational anesthetics (halothane, isoflurane & enflurane) may be indicated in refractroy case 64
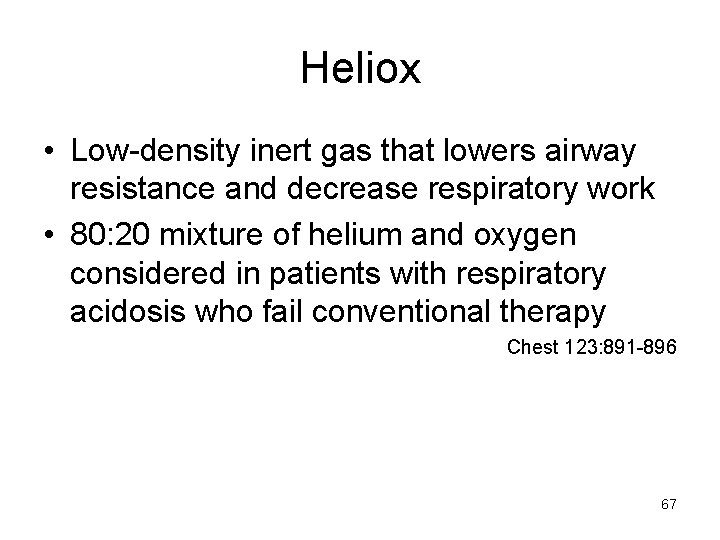
Heliox • Low-density inert gas that lowers airway resistance and decrease respiratory work • 80: 20 mixture of helium and oxygen considered in patients with respiratory acidosis who fail conventional therapy Chest 123: 891 -896 67
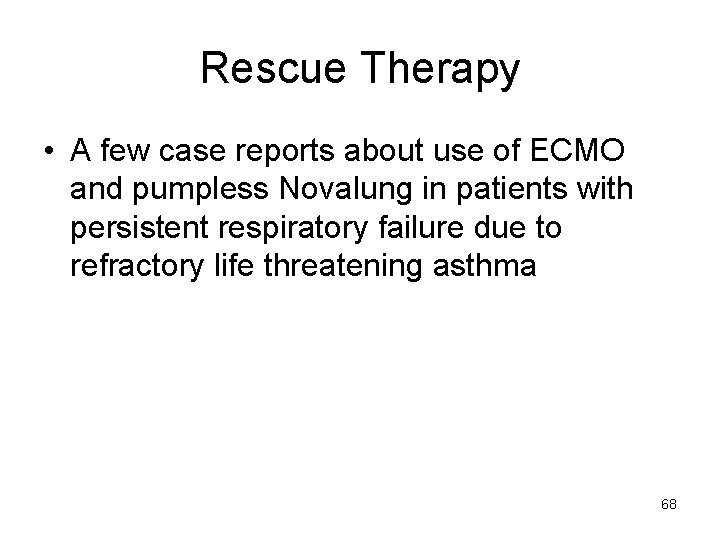
Rescue Therapy • A few case reports about use of ECMO and pumpless Novalung in patients with persistent respiratory failure due to refractory life threatening asthma 68
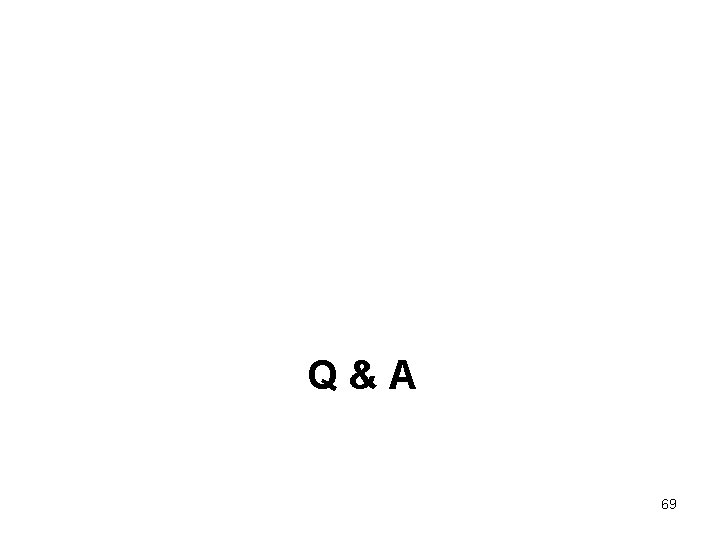
Q&A 69
Pathophysiology definition
Complications of asthma
Status asthmaticus
Mechanical ventilator modes
Purpose of mechanical ventilation
Transpulmonary pressure formula
Dope mechanical ventilation
332 rule intubation
Complications of mechanical ventilation
Pressure support ventilation
Mechanical ventilation learning package
Mechanical ventilation definition
Pressure support ventilation ppt
Types of ventilation fire fighting
Jenny tsang-quinn
Ooi wei tsang
Sharing
Dr peter tsang
Jody tsang
Gabriel tsang
Dr karen shiu
Martin wills warwick
Dr. peter tsang
Actual mechanical advantage vs ideal mechanical advantage
Pressure support ventilation
Respiration vs ventilation
Industrial ventilation engineering principles
Types of ventilator modes
Aprv waveform
As 91155
Ventilation
Residential ventilation fans market
Pulmonary ventilation
Atelectrauma
Ubbl sprinkler system
Cpap for dummies
Ventilation calculation formula for confined space
Vertical ventilation definition
Prvc disadvantages
Bag mask ventilation
Vc+ vs prvc
Simv
Industrial ventilation hood
Office administration system
"hw ventilation"
Pulse check in unresponsive victim
Pulmonary ventilation
East coast ventilation
Natural ventilation
Respiratory
How do you calculate ventilation rate
Ventilation sdb
Balanced ventilation example
Dynamic compliance
Industrial ventilation engineering principles
Todd ring
T-piece ventilation
Norwani ahmat
Pression crete valeur normale
Ventilation
General ventilation
Non invasive ventilation
Dyspneic index formula
Principles of air conditioning ppt
Non invasive ventilation
God ventilation
Infant cpr algorithm
Ventilation artificielle