Mark Lycett Ventilation Mechanical Ventilation Mechanical Ventilation Aims
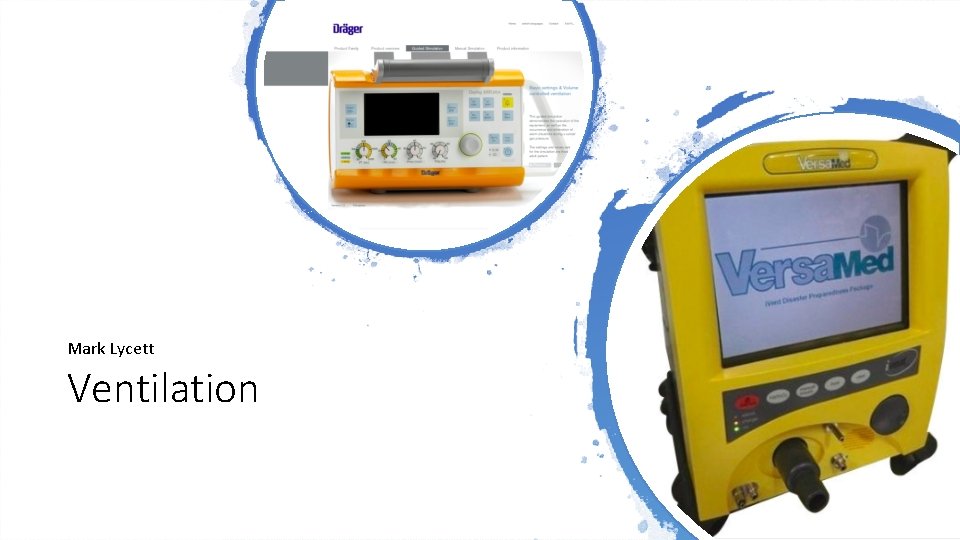
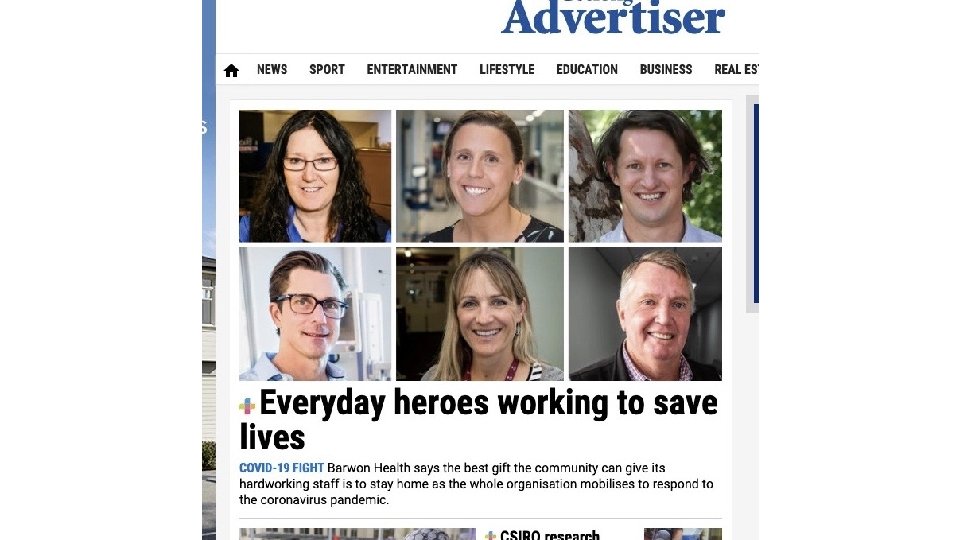
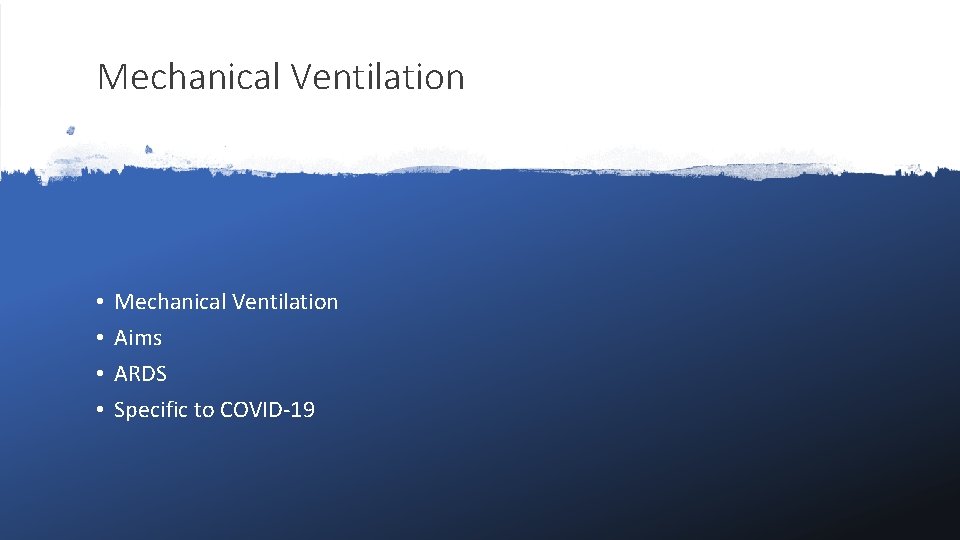
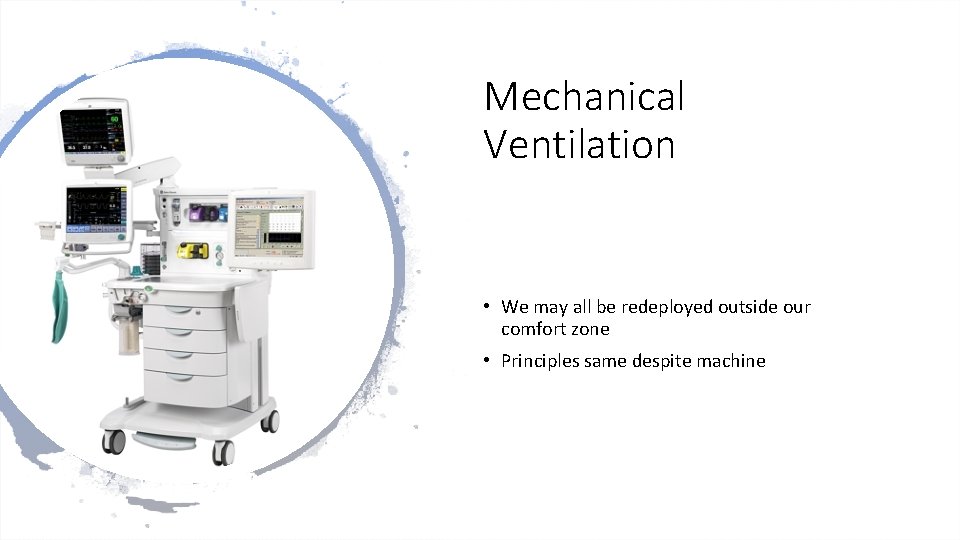
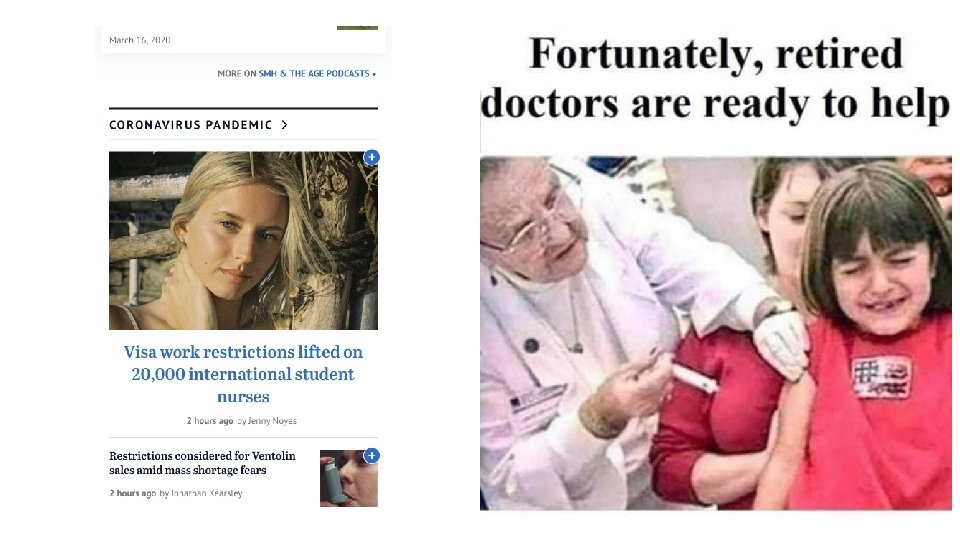
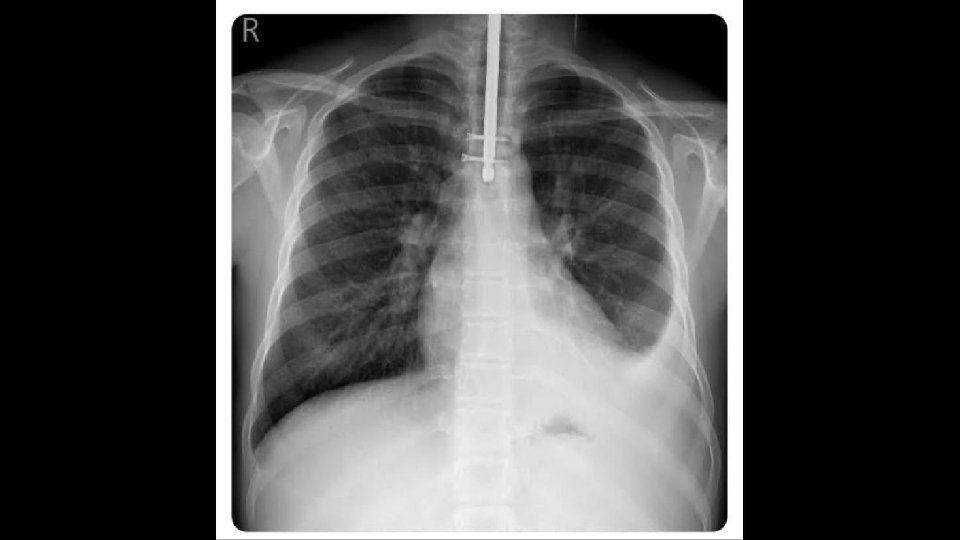
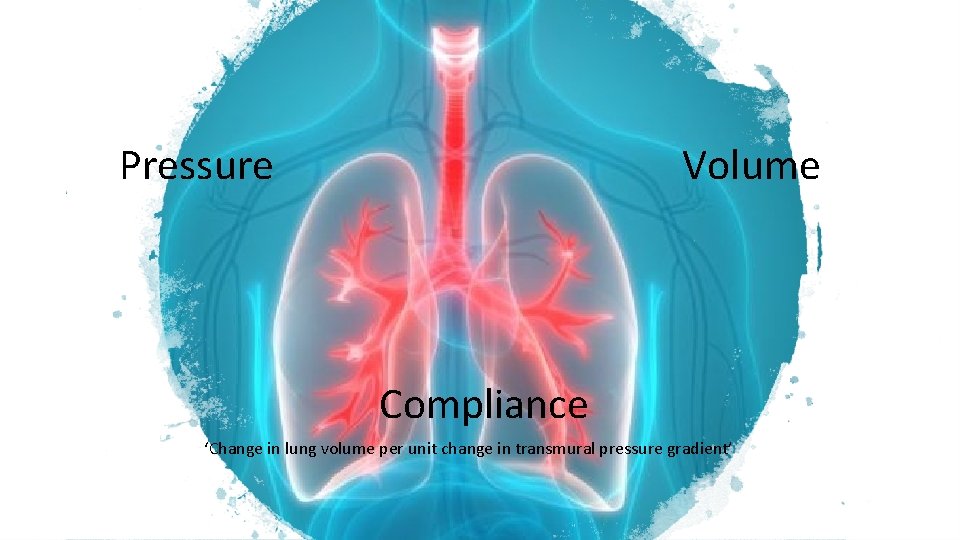
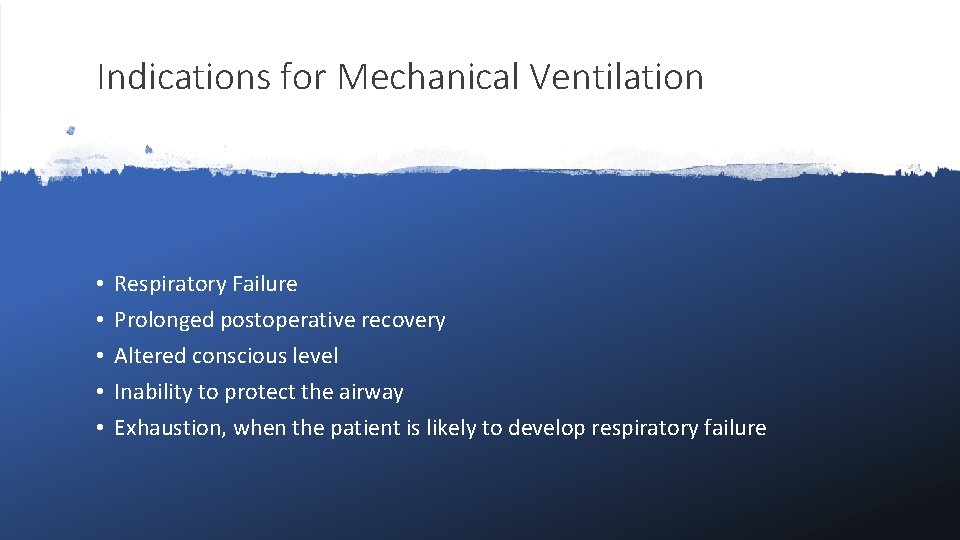
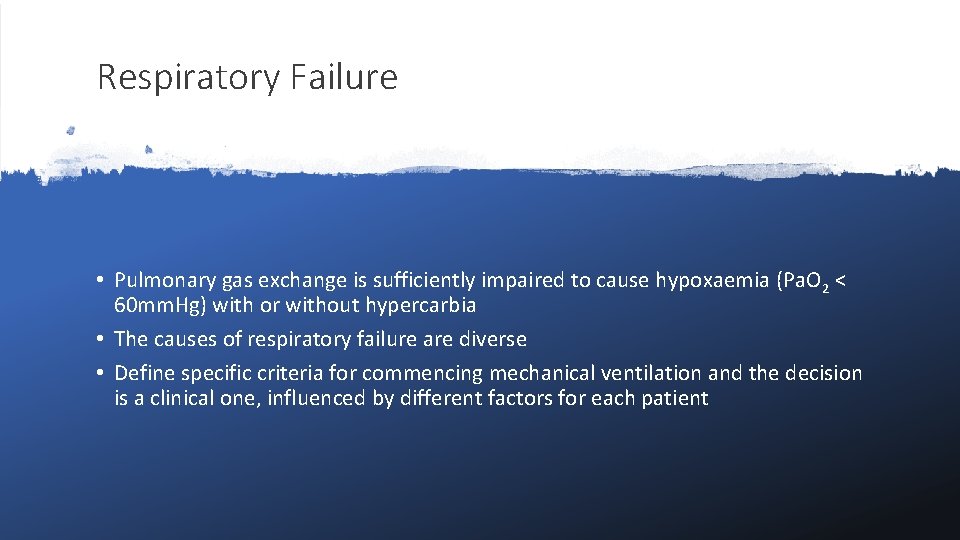
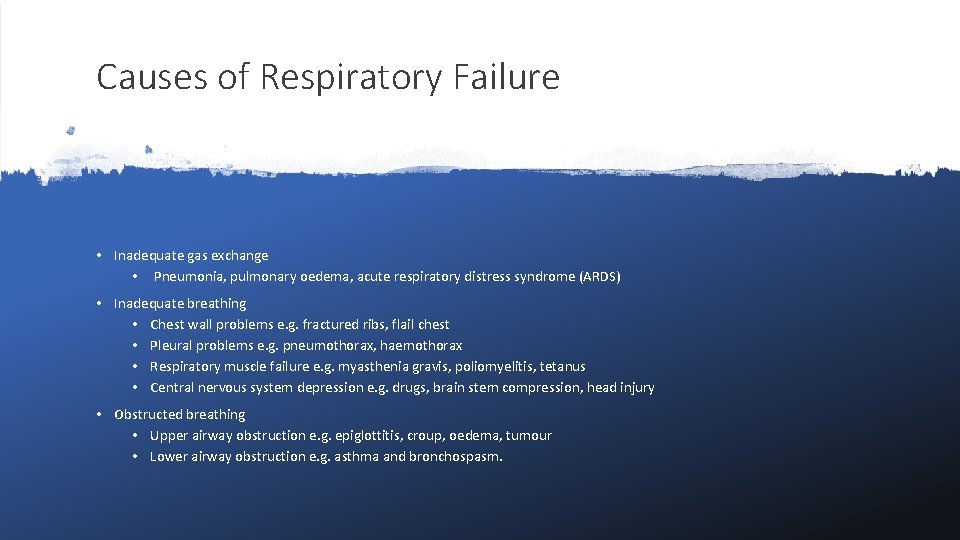
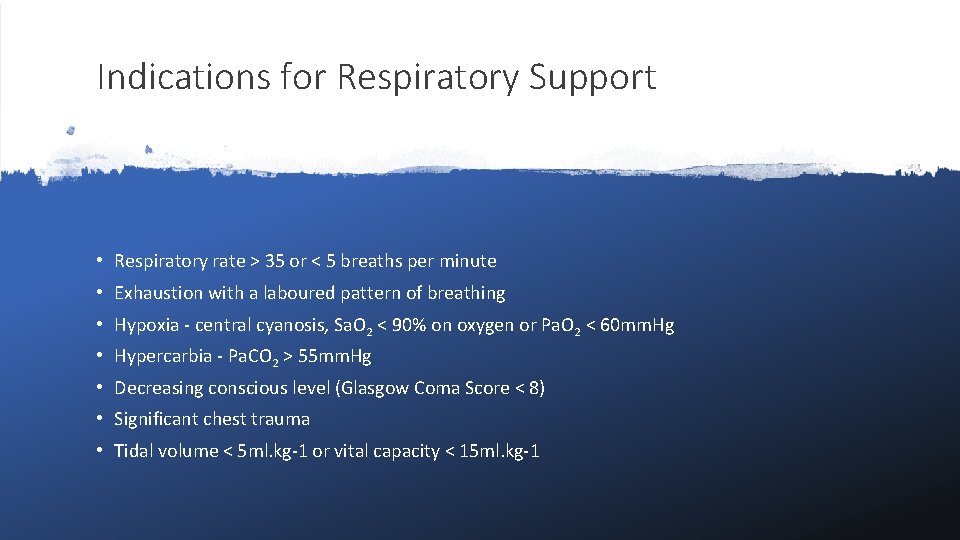
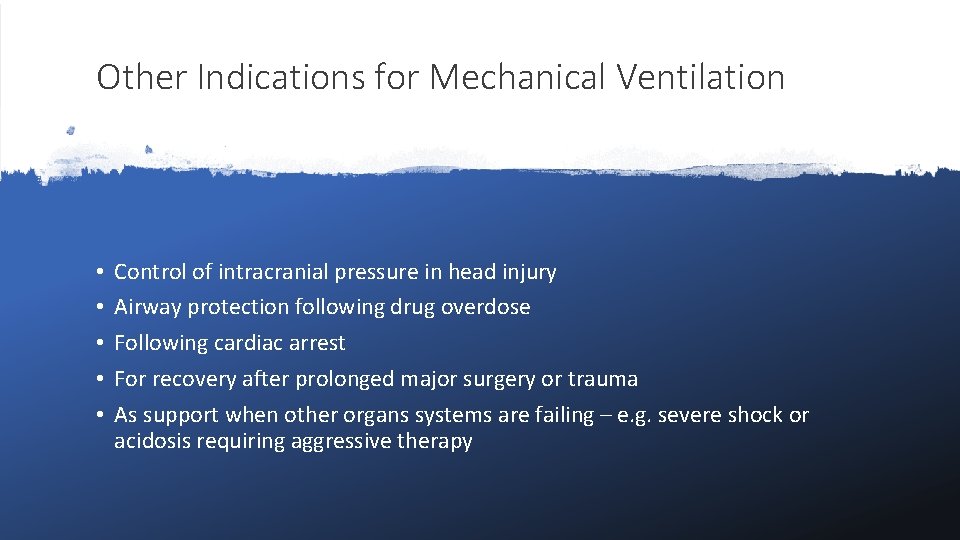
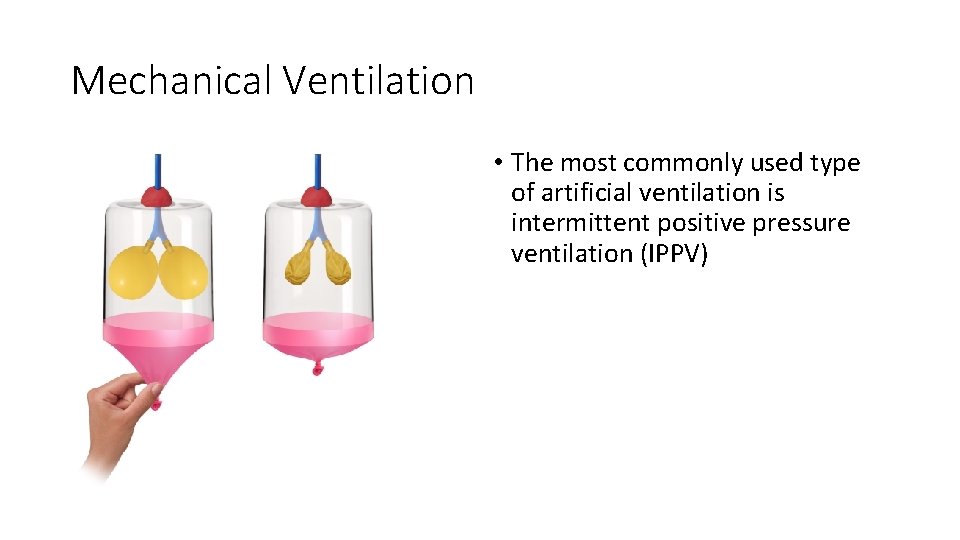
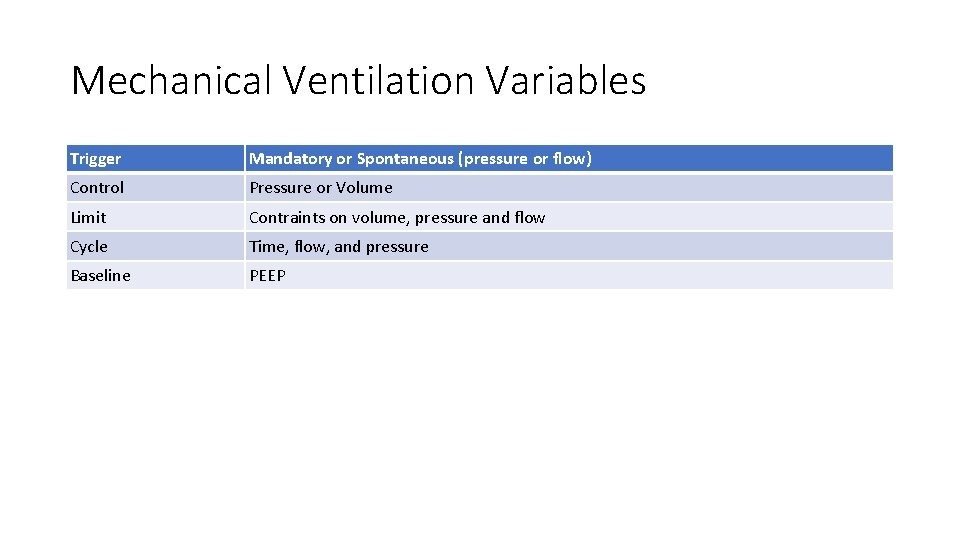
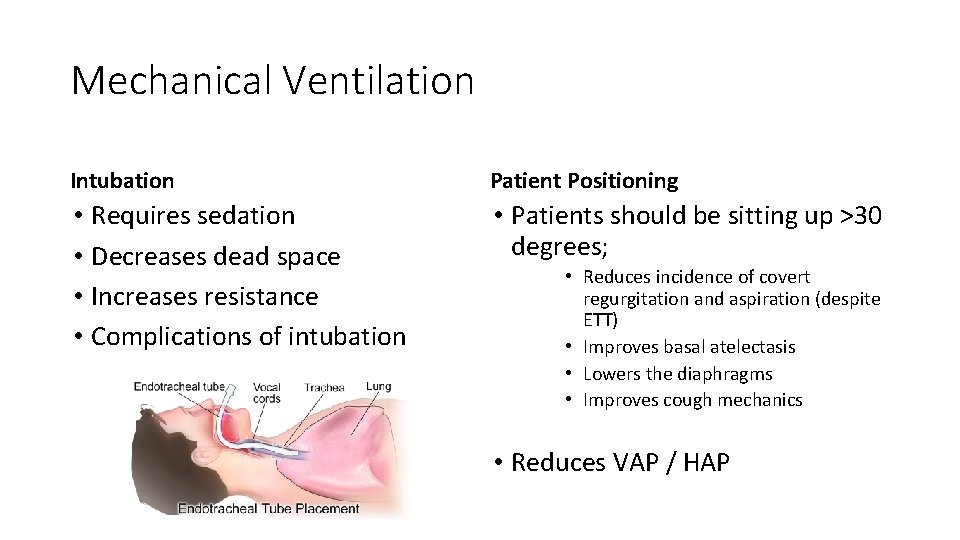
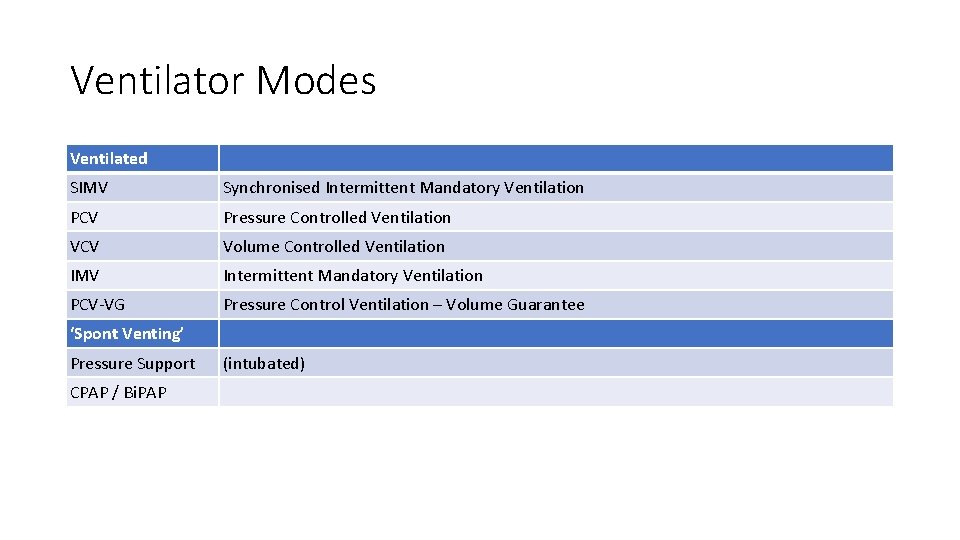
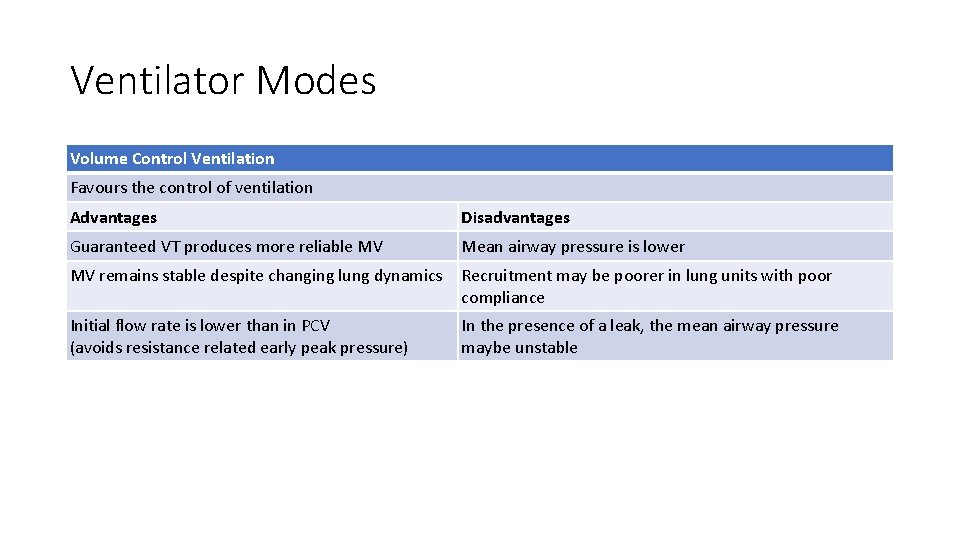
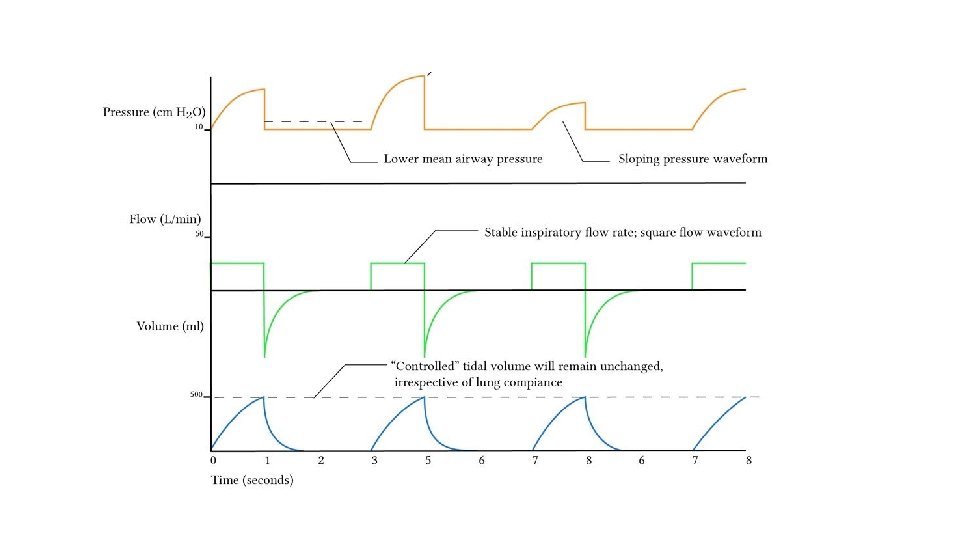
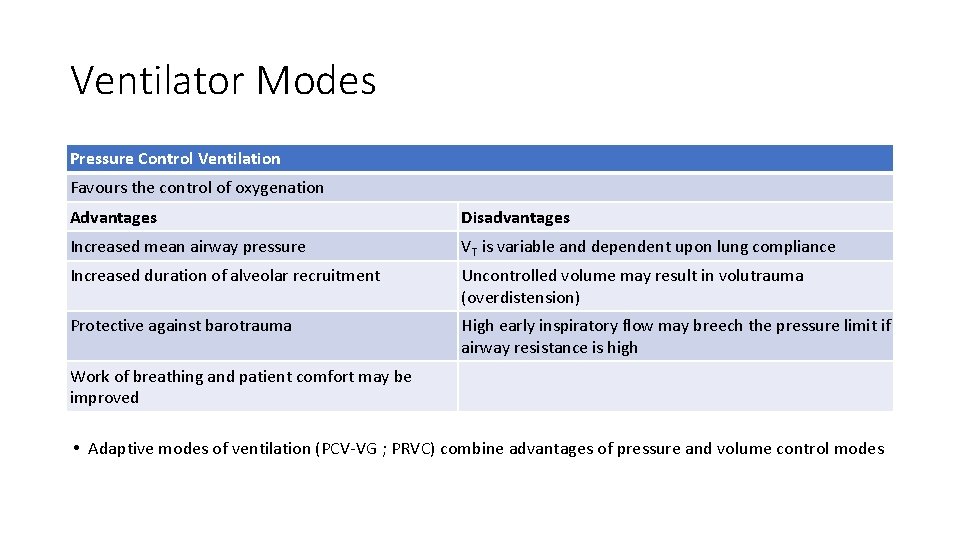
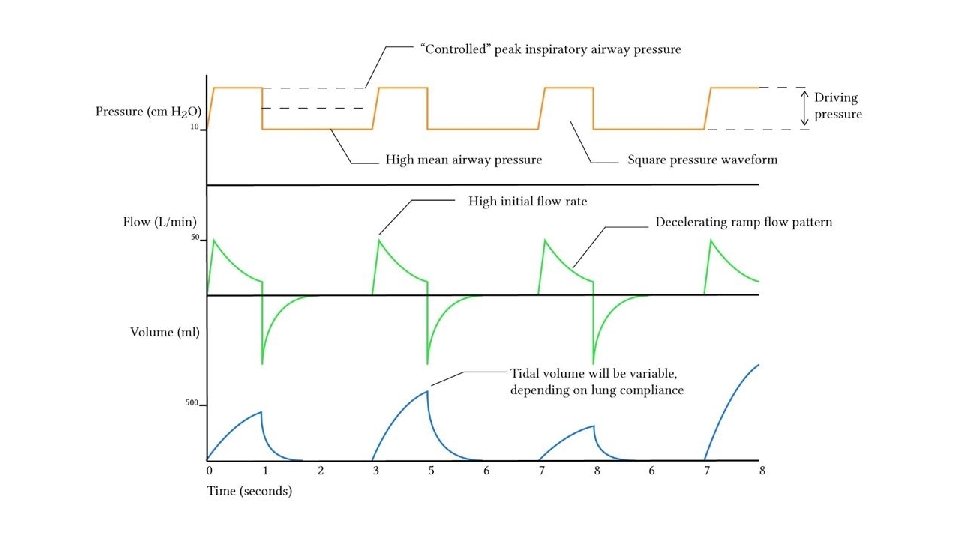
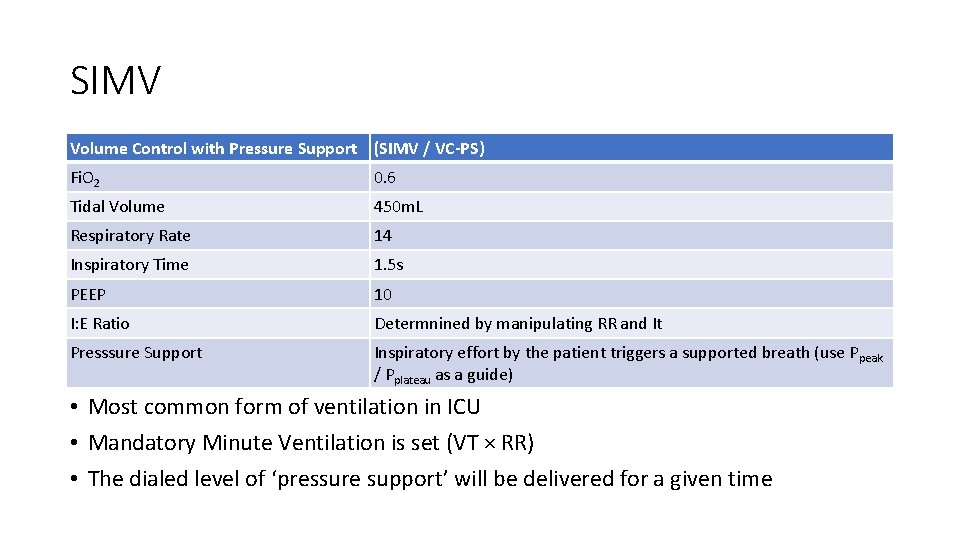
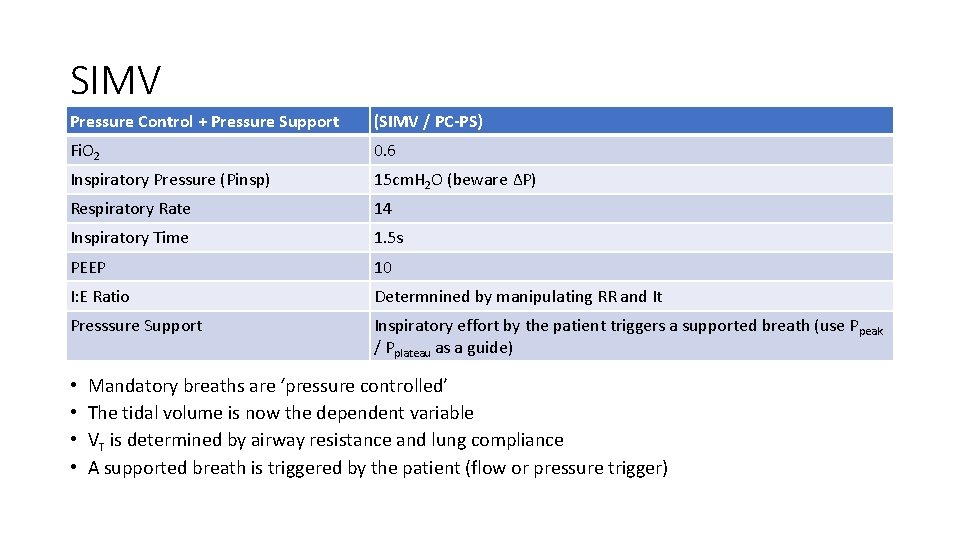
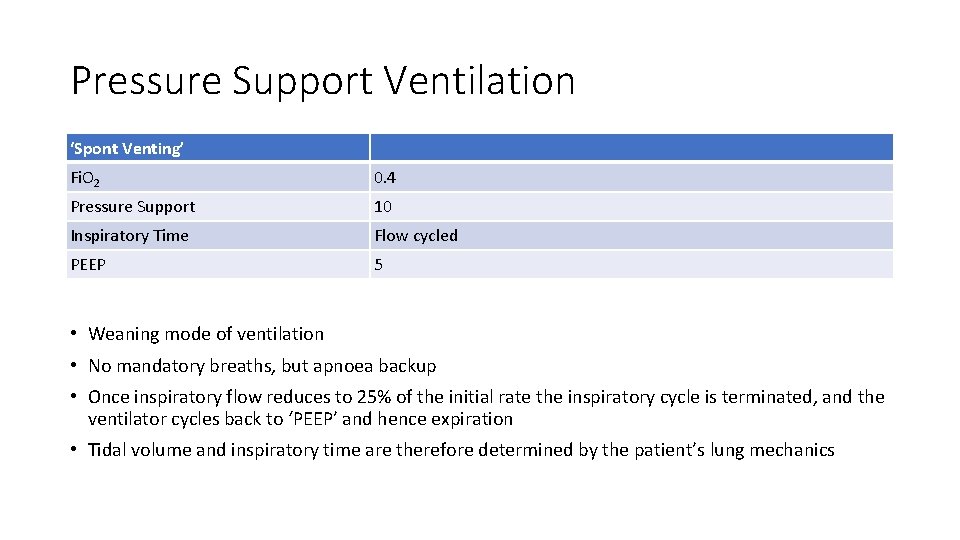
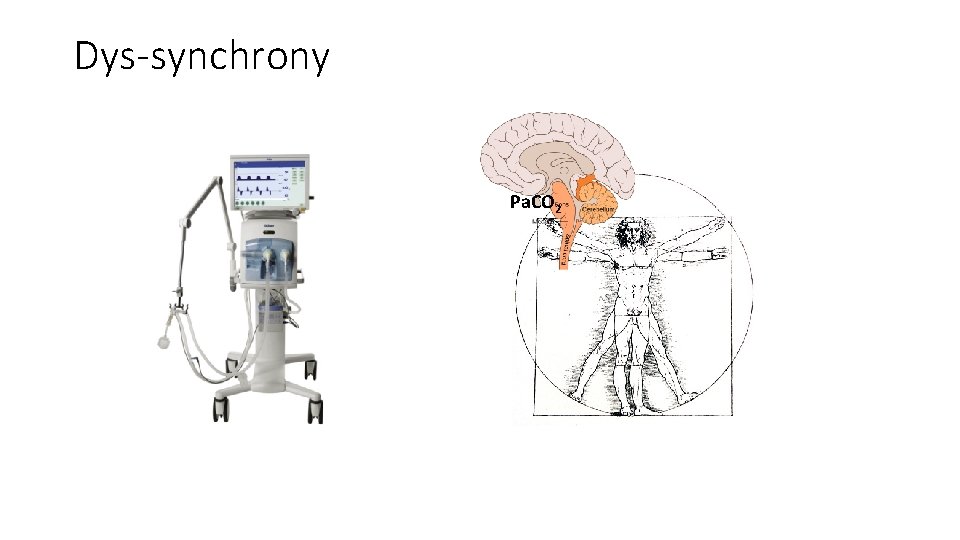
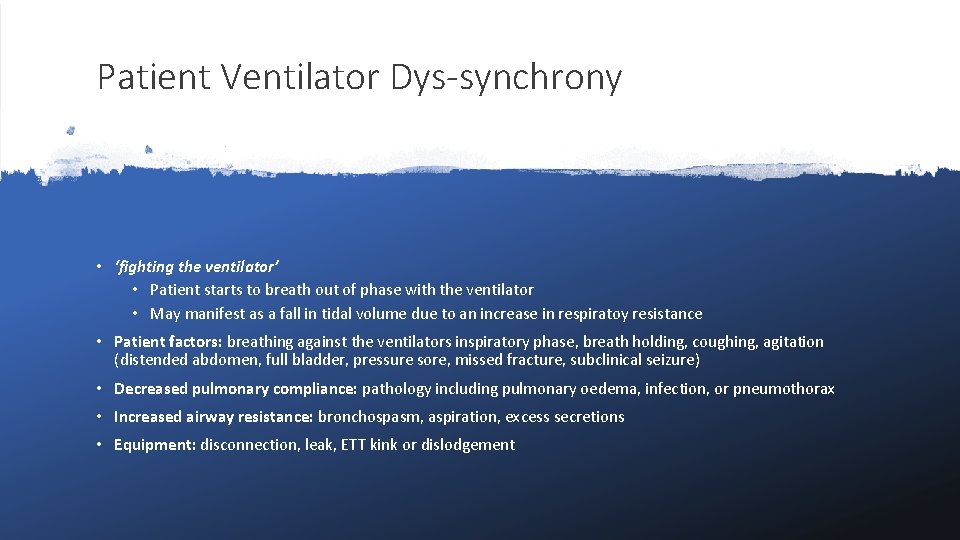
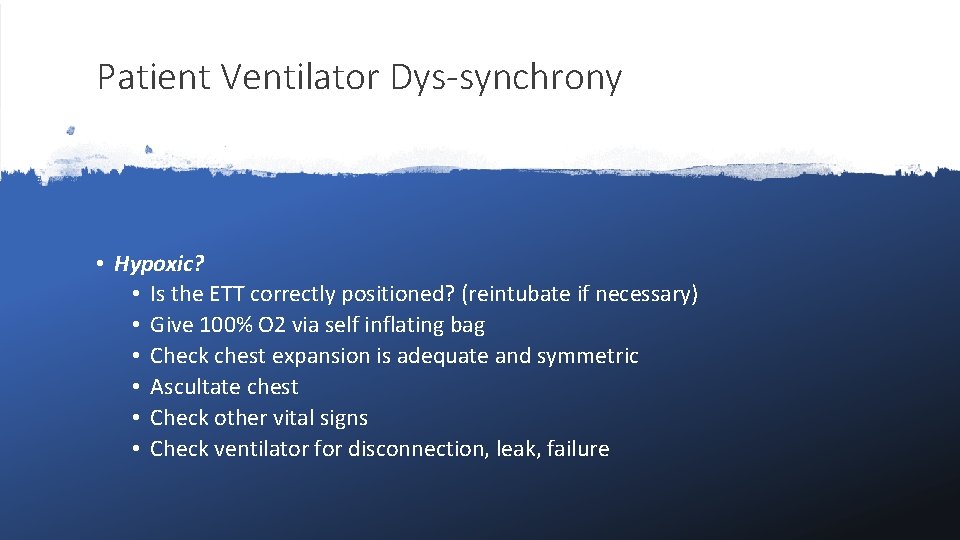
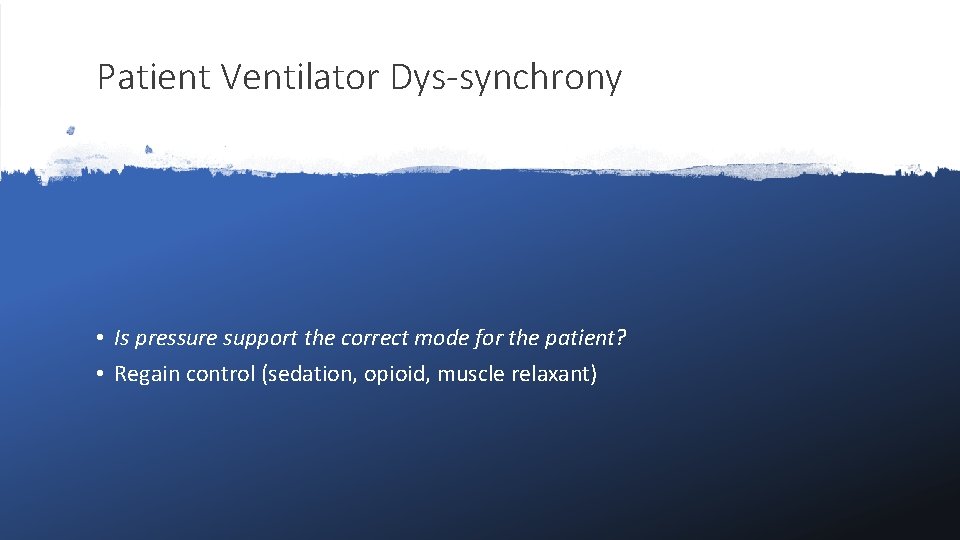
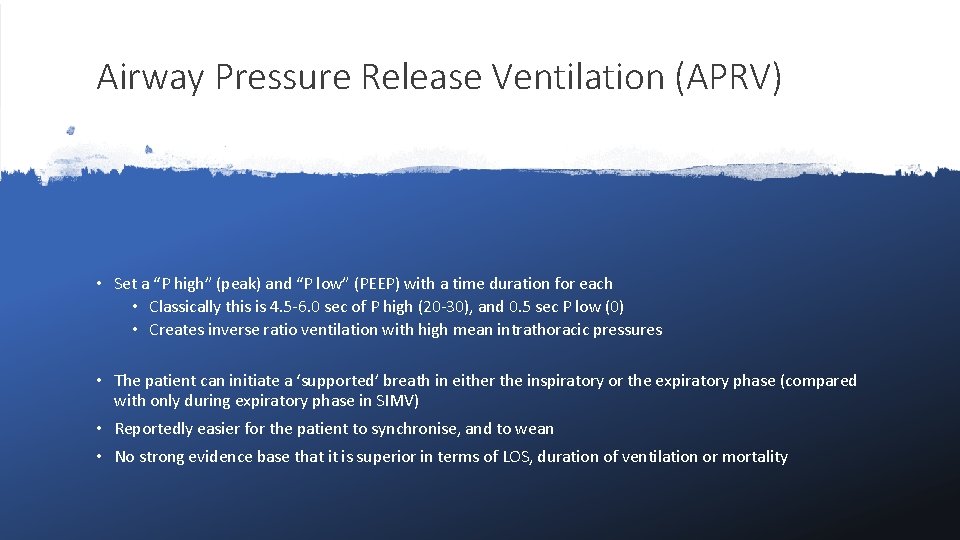
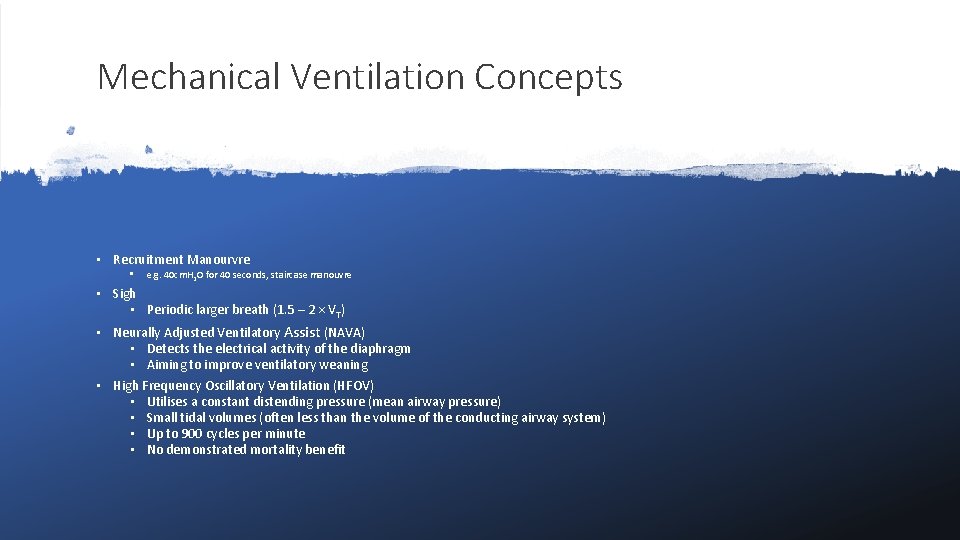
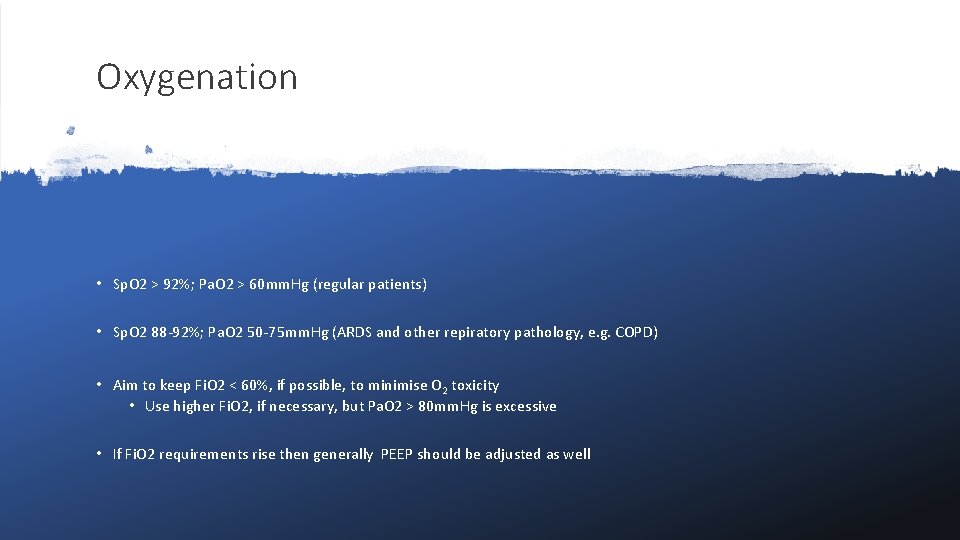
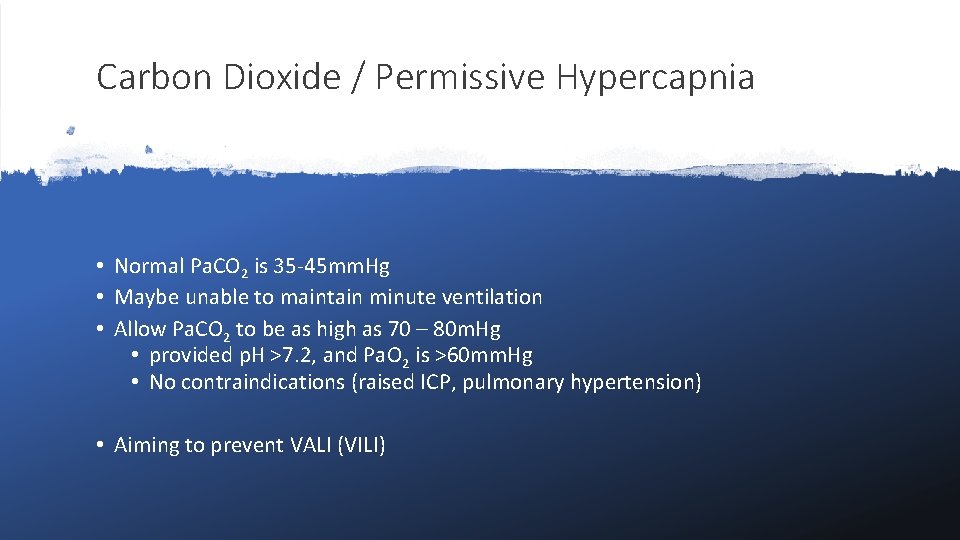
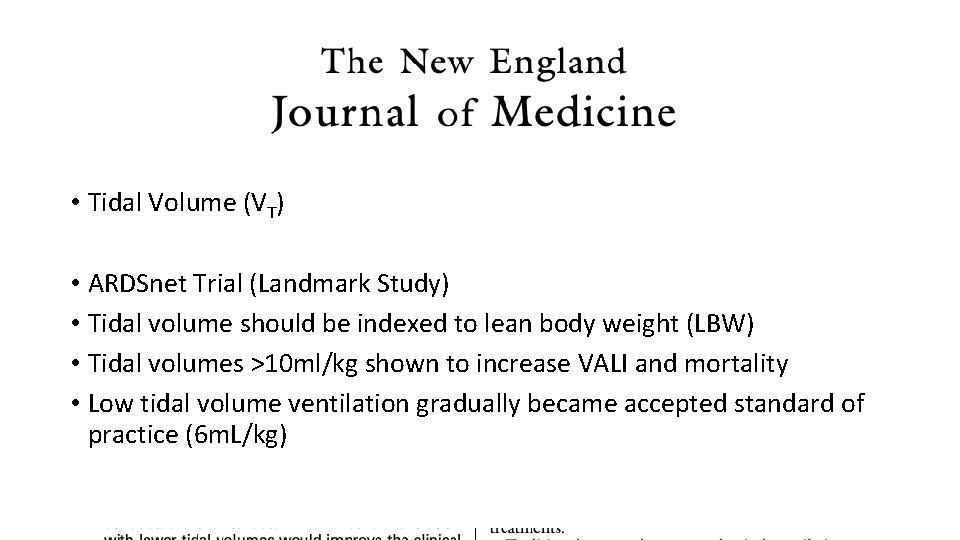
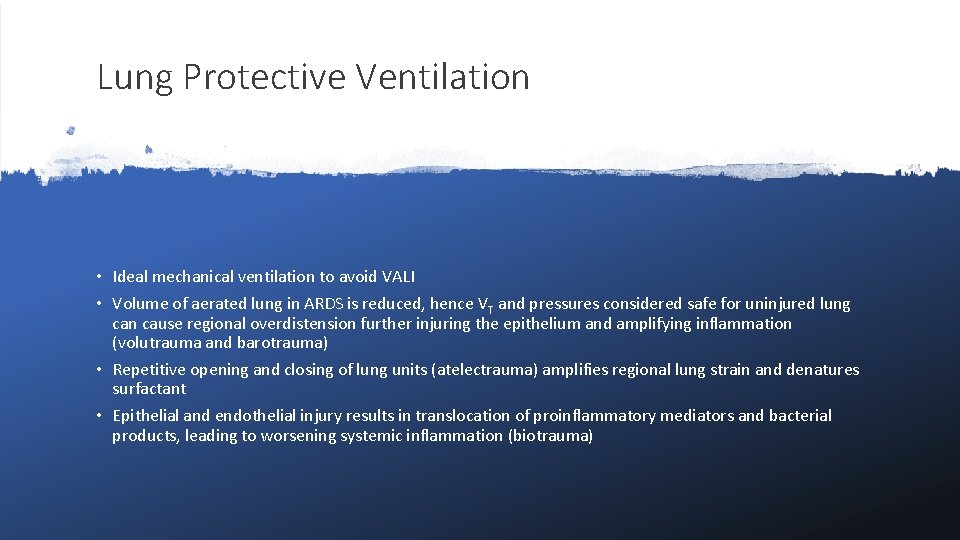
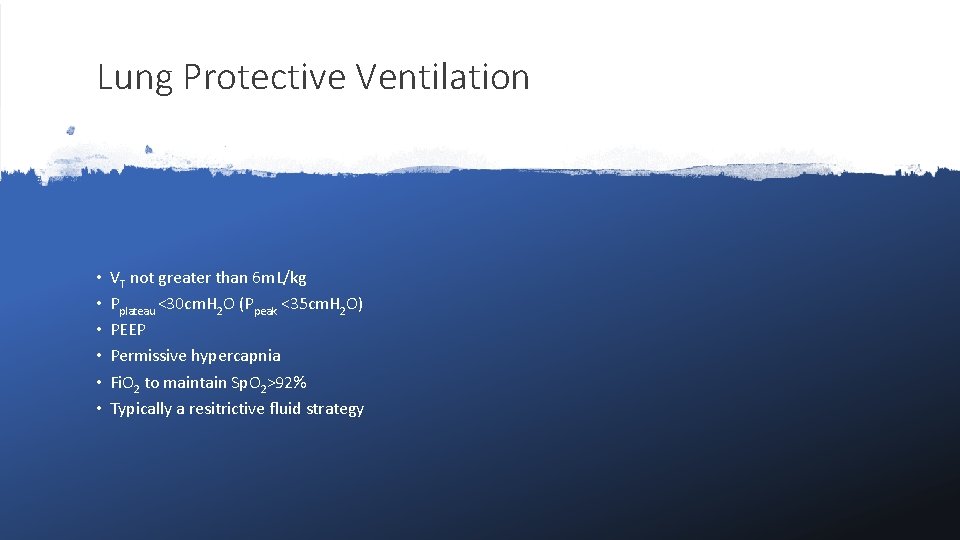
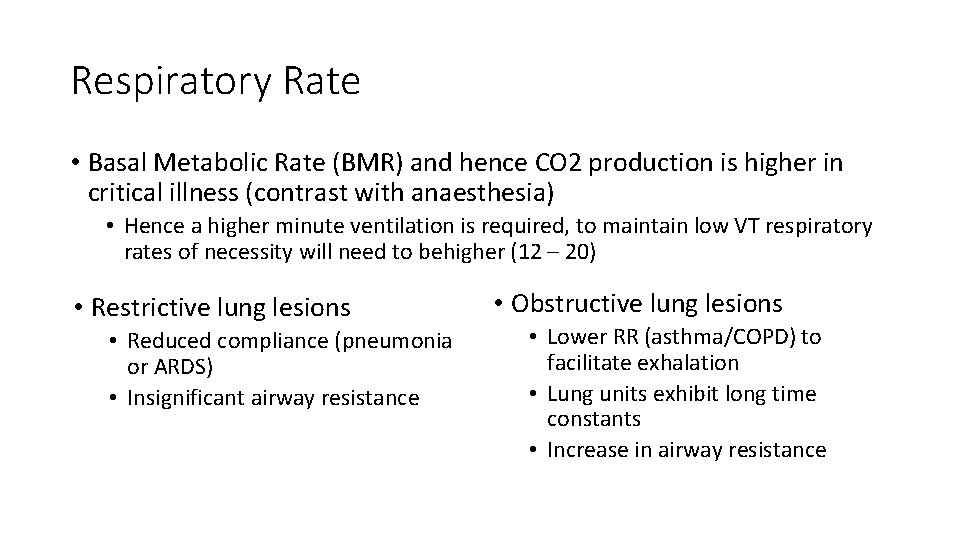
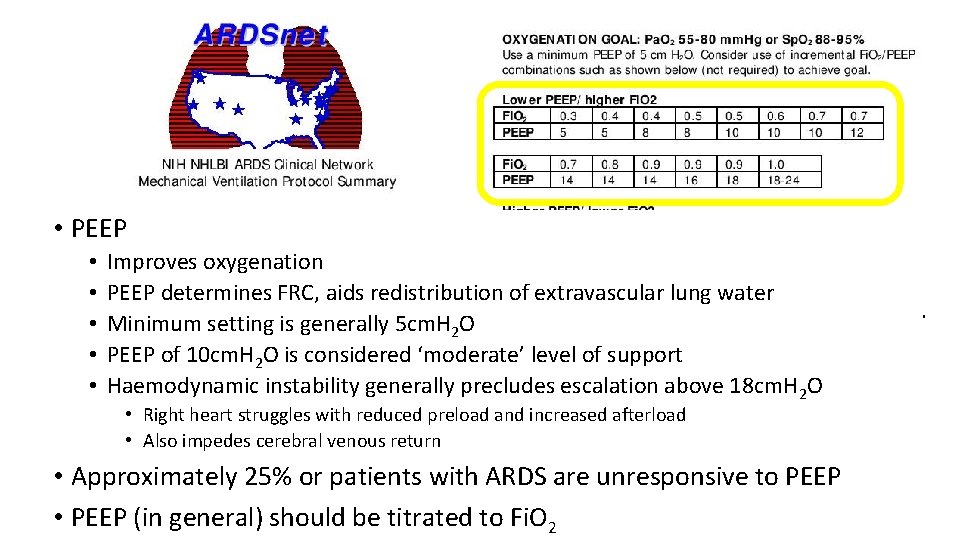
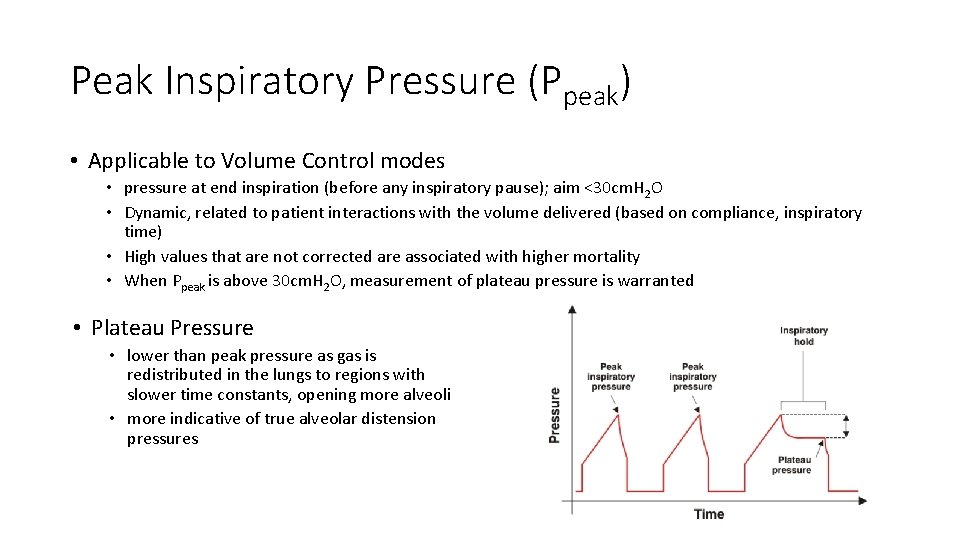
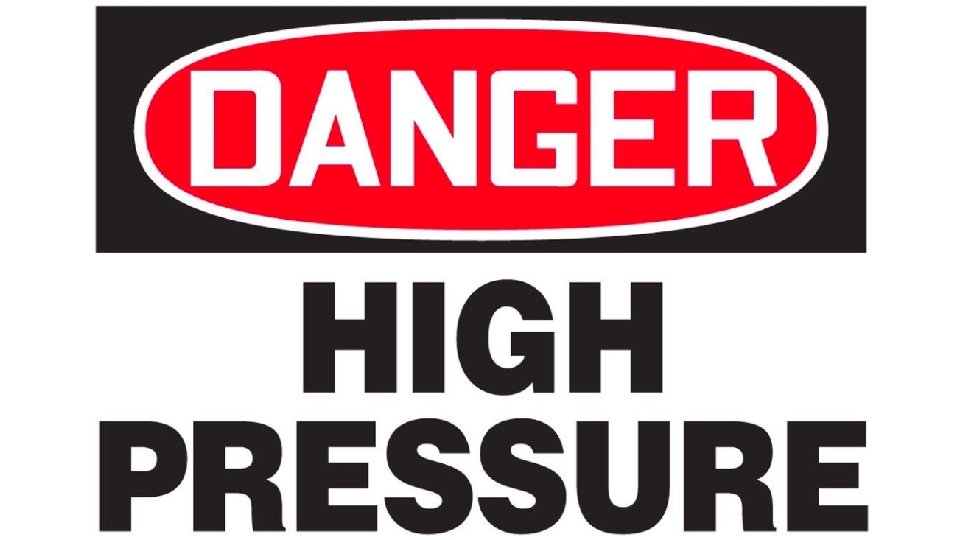
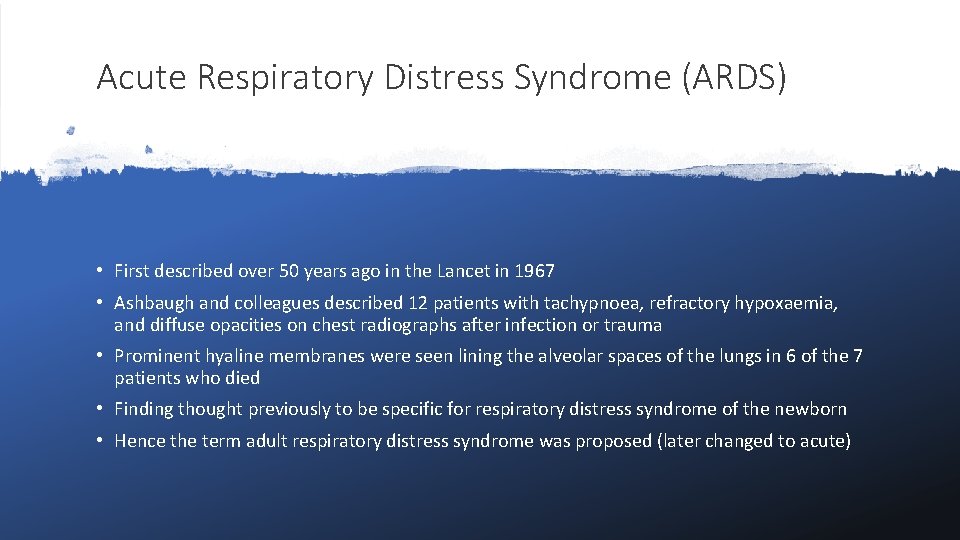
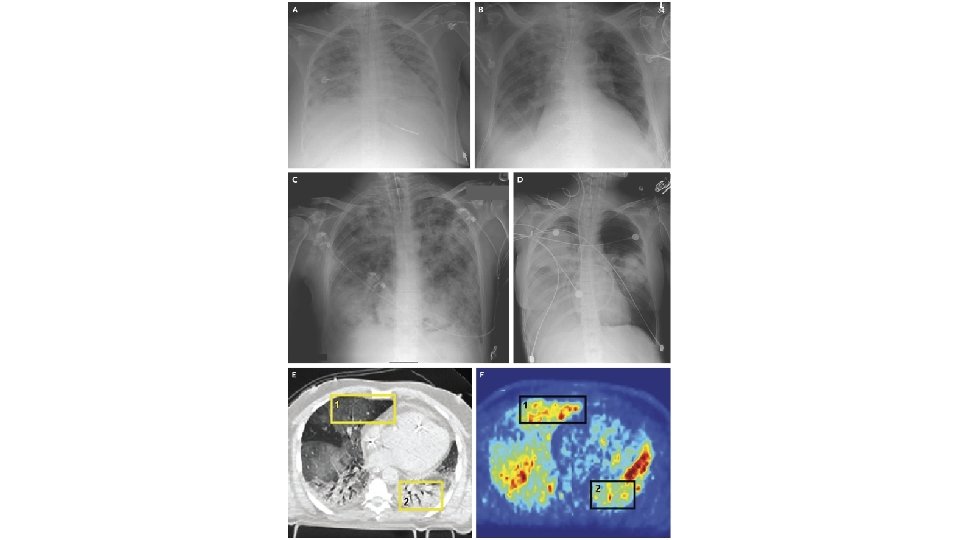
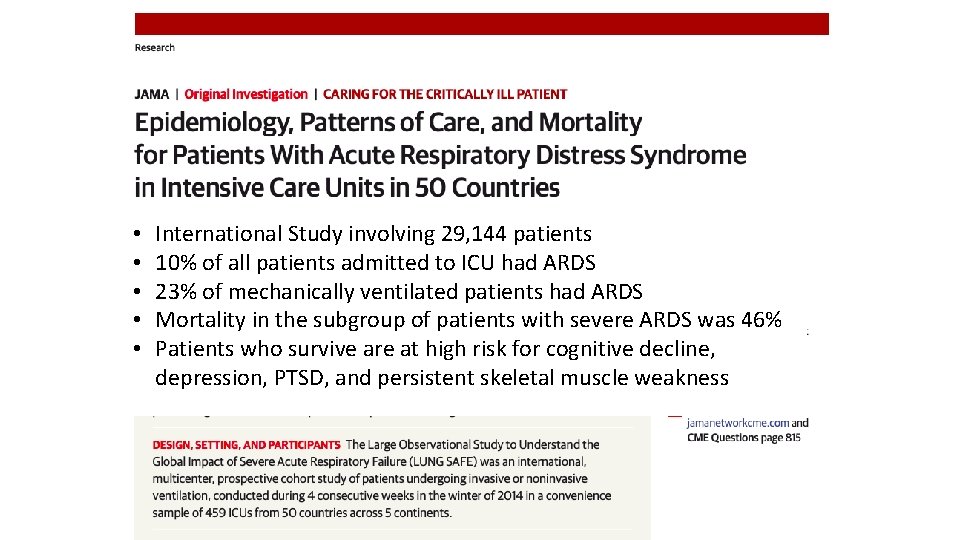
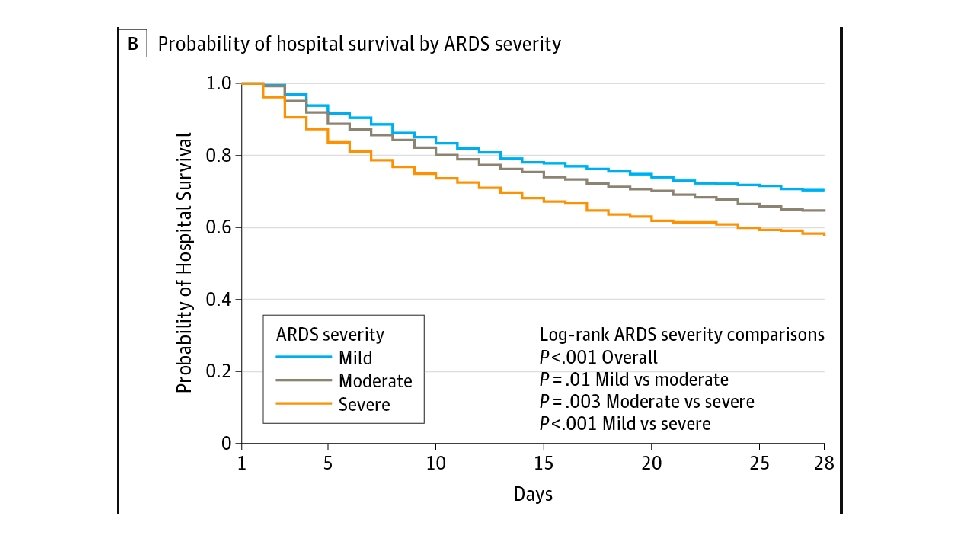
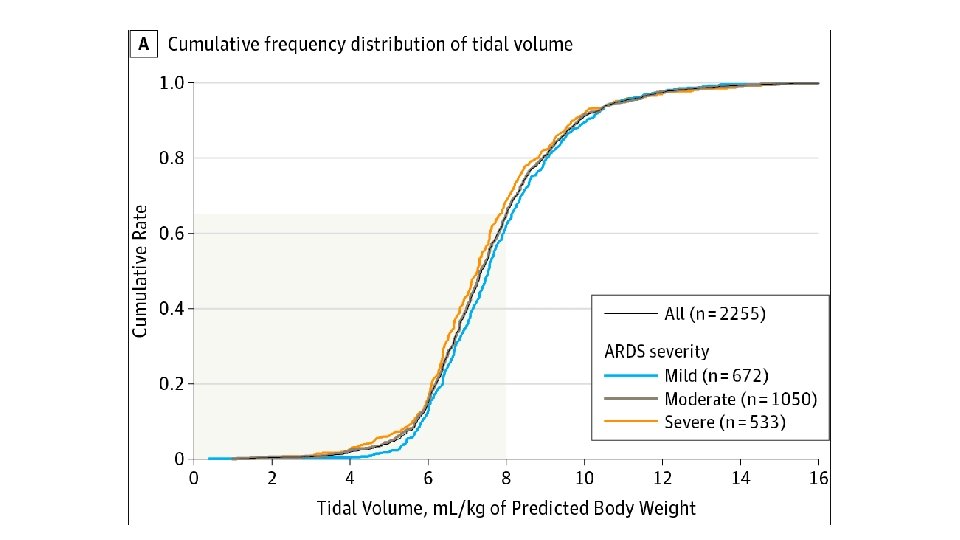
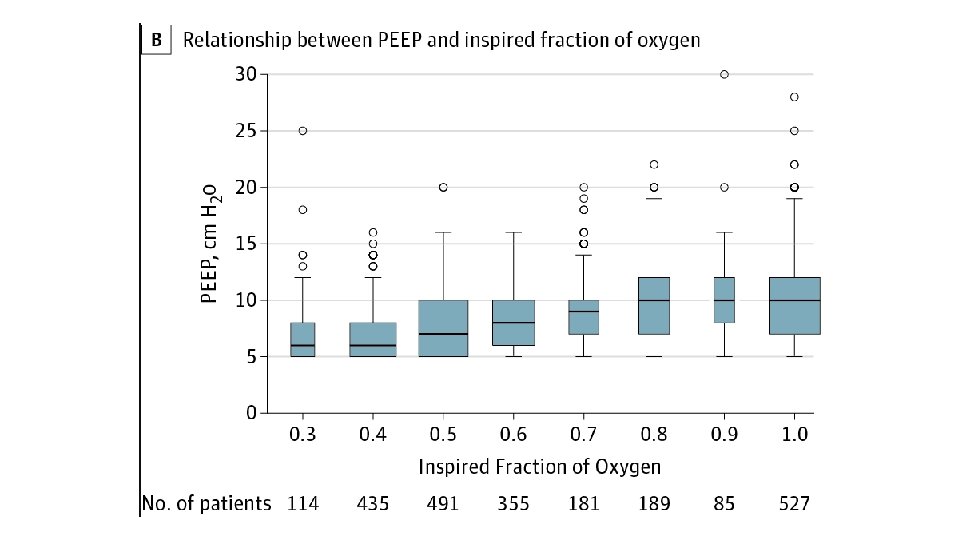
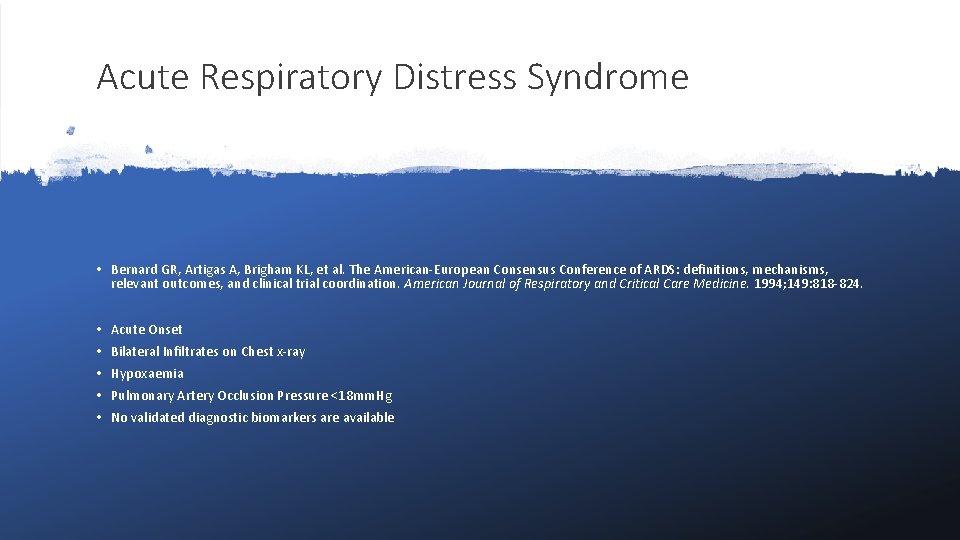
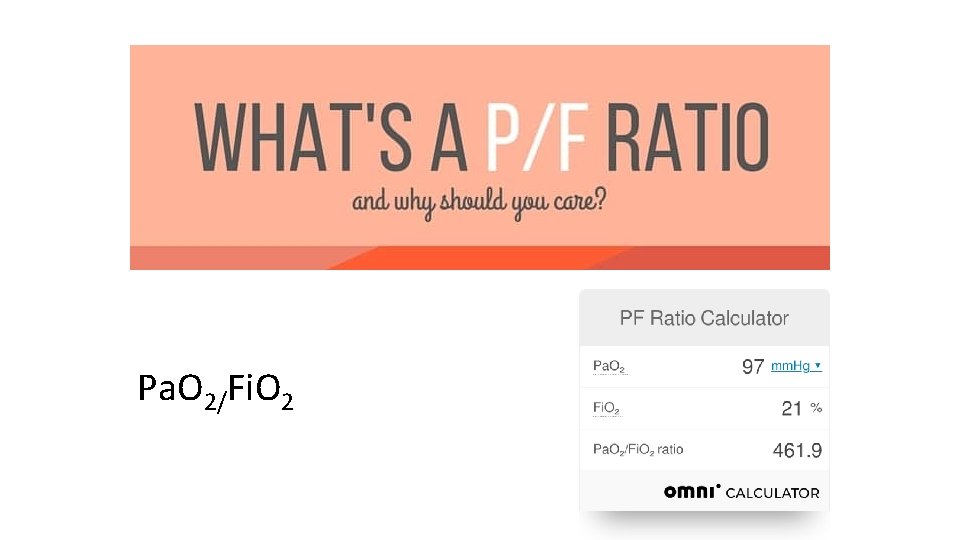
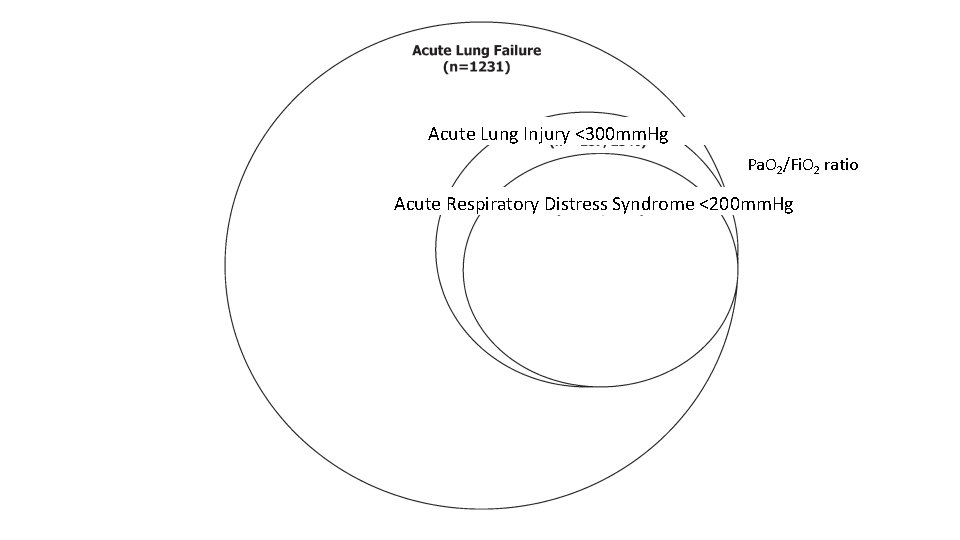
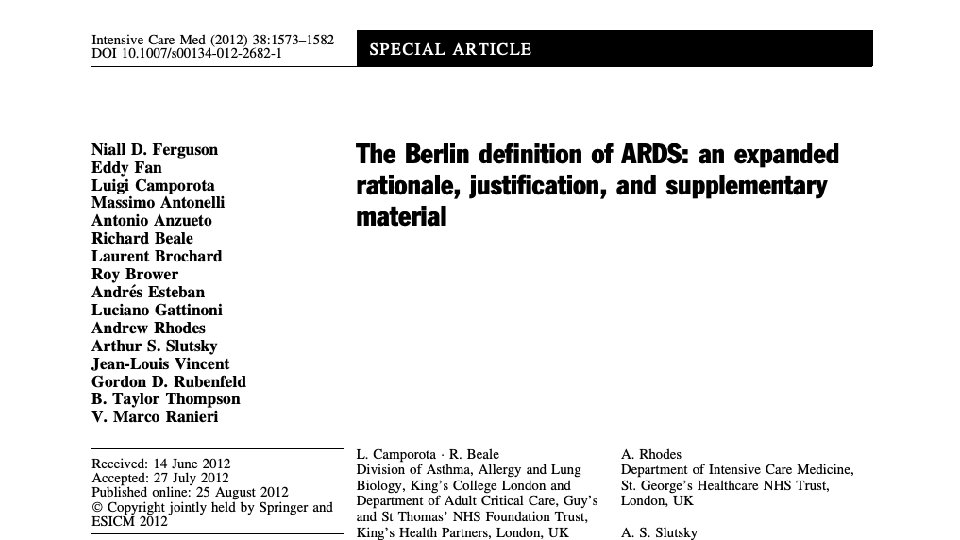
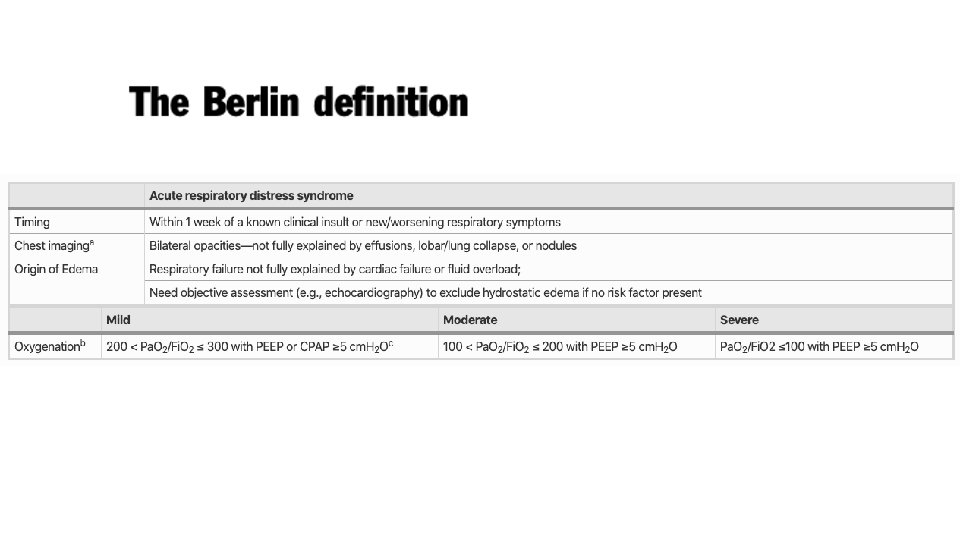
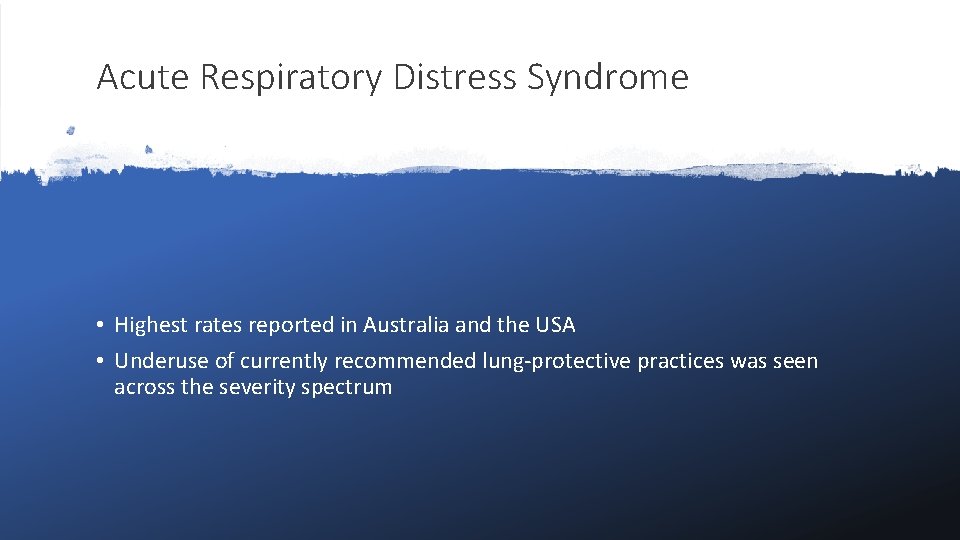
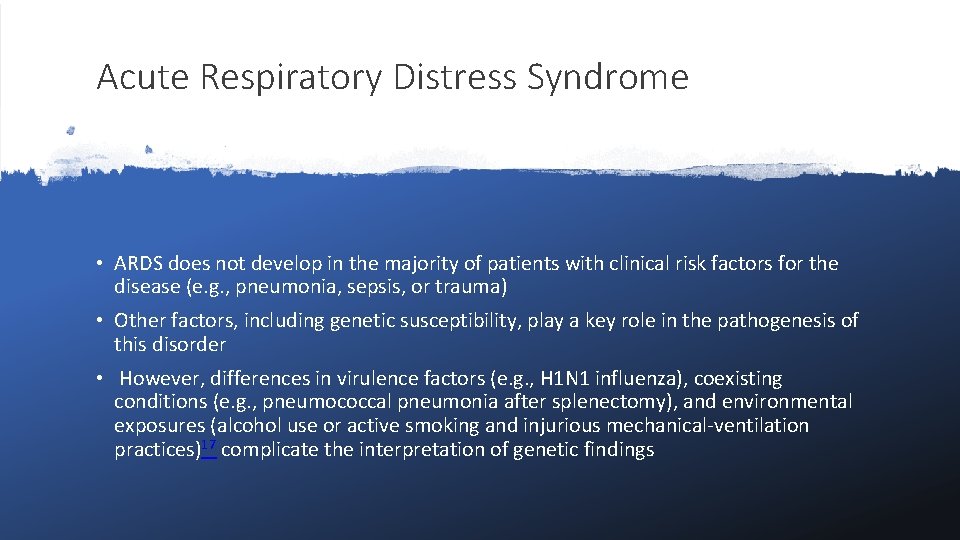
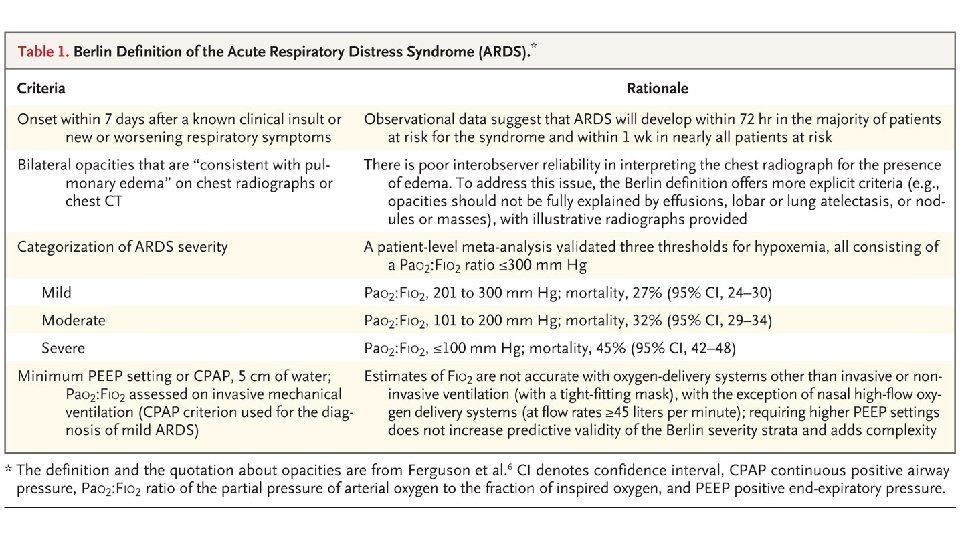
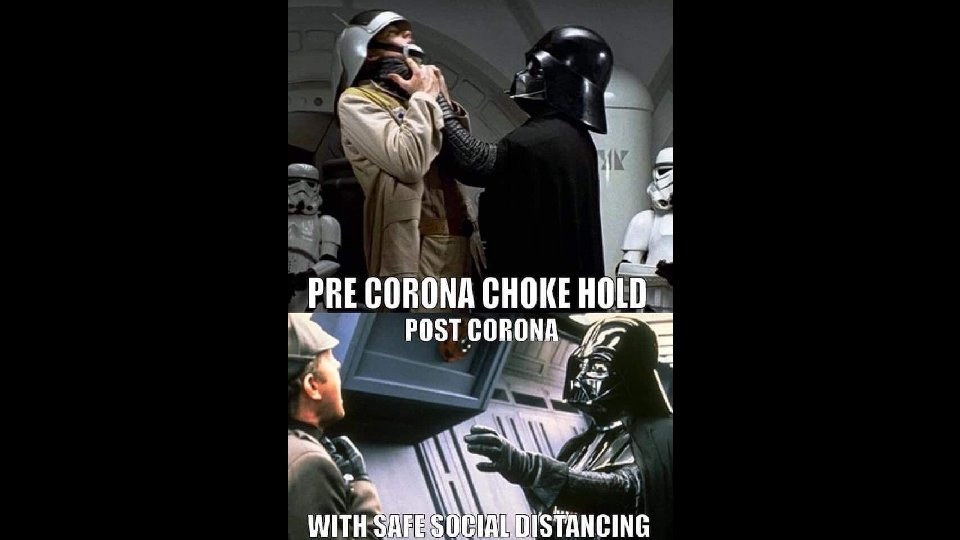
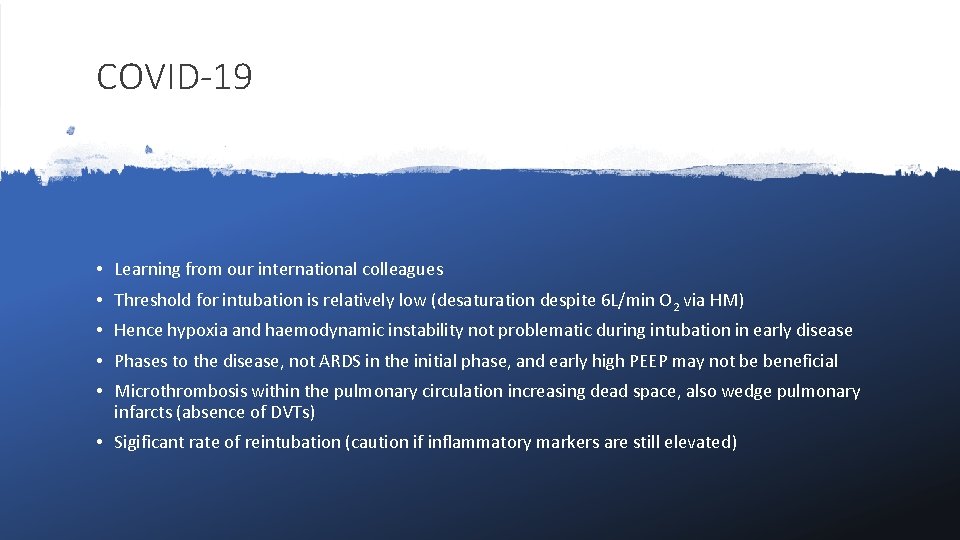
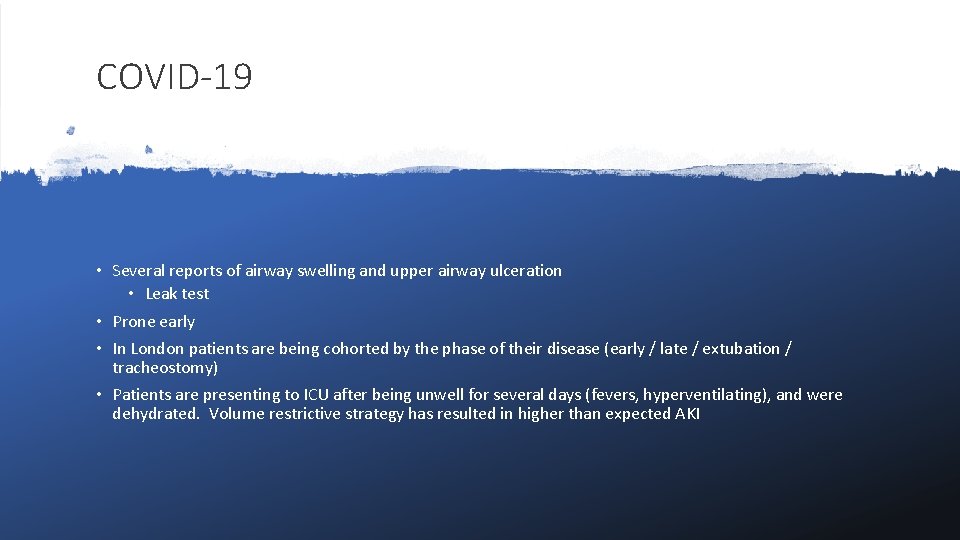
- Slides: 55
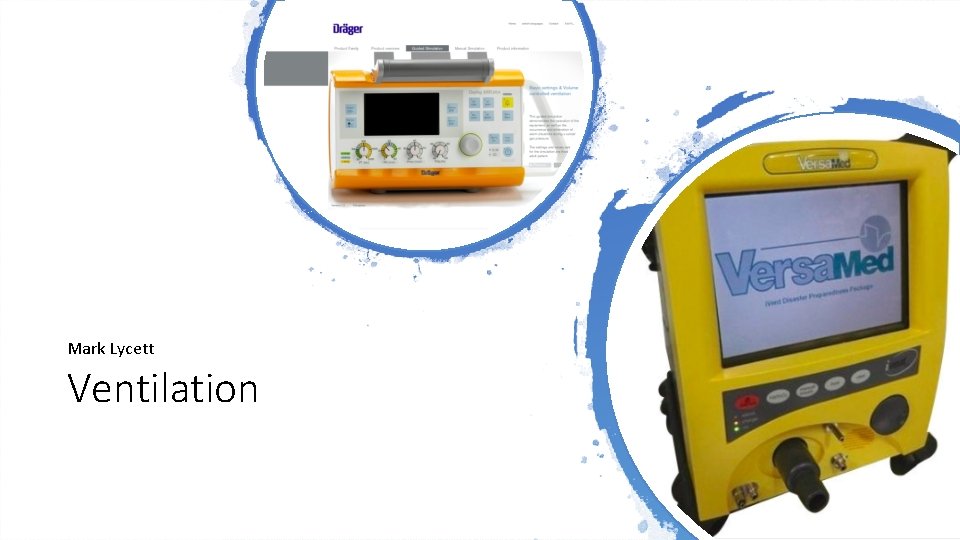
Mark Lycett Ventilation
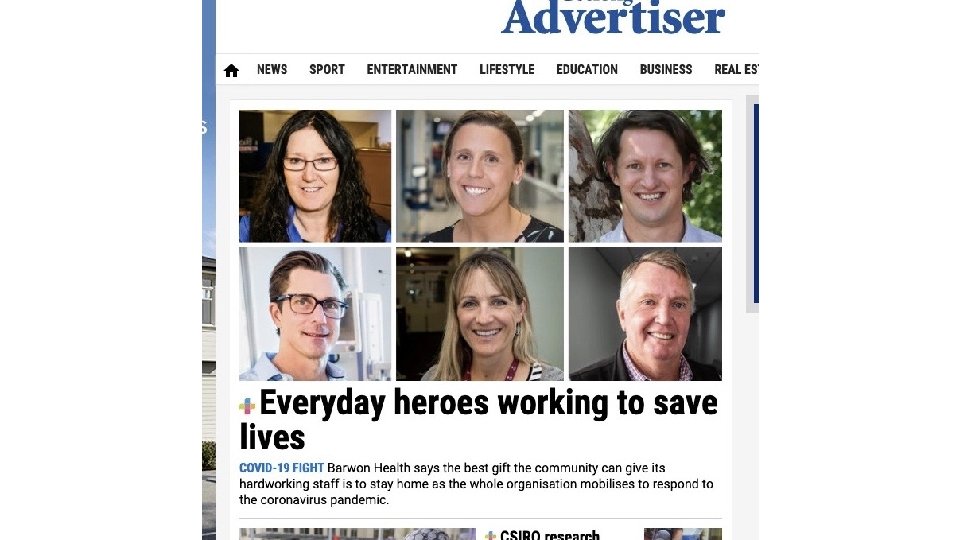
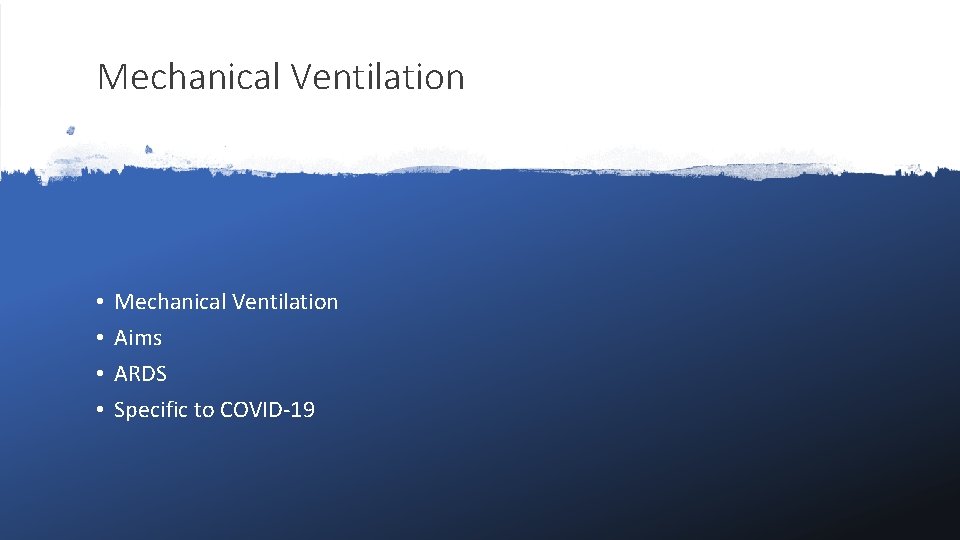
Mechanical Ventilation • • Mechanical Ventilation Aims ARDS Specific to COVID-19
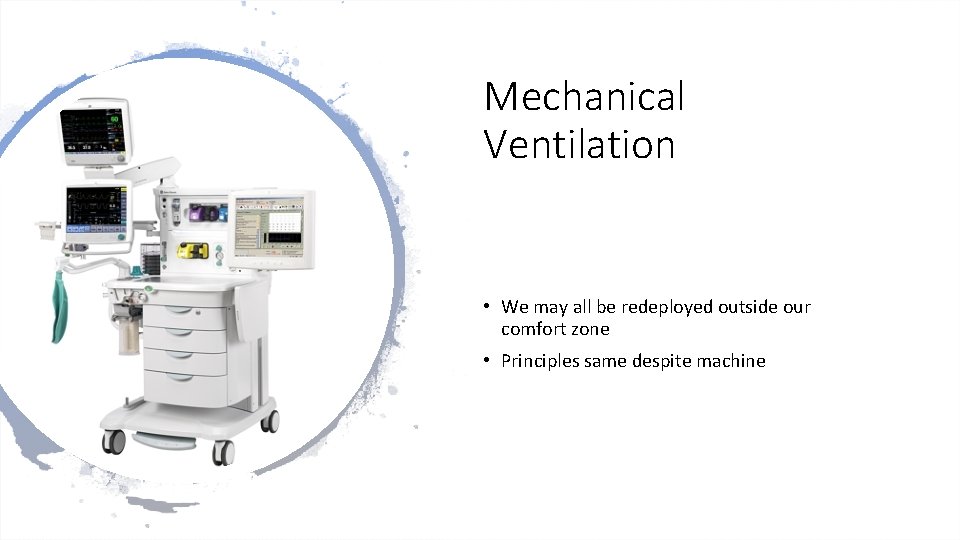
Mechanical Ventilation • We may all be redeployed outside our comfort zone • Principles same despite machine
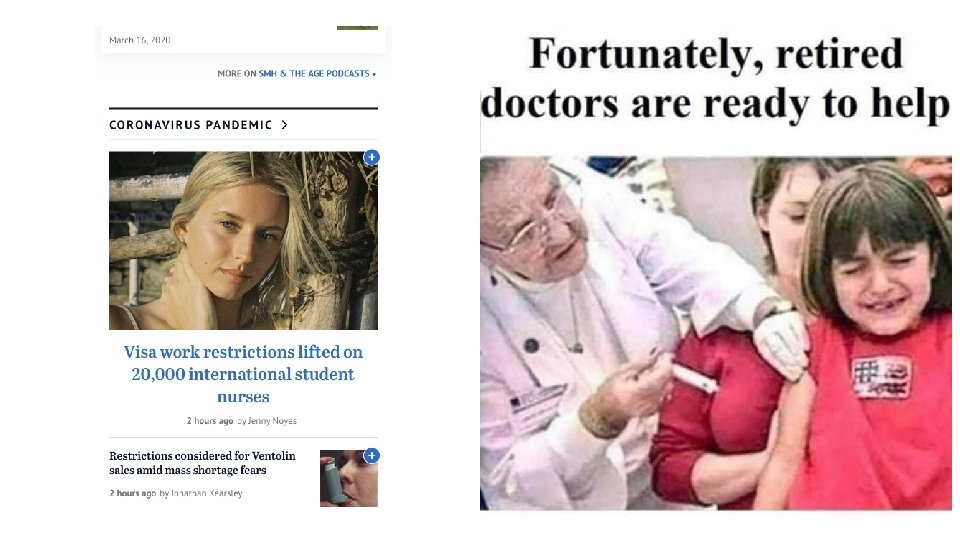
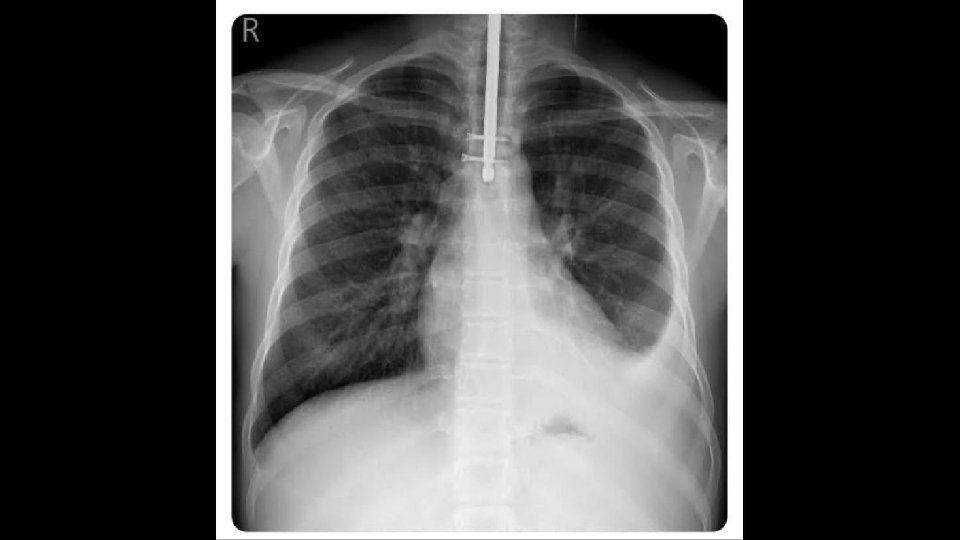
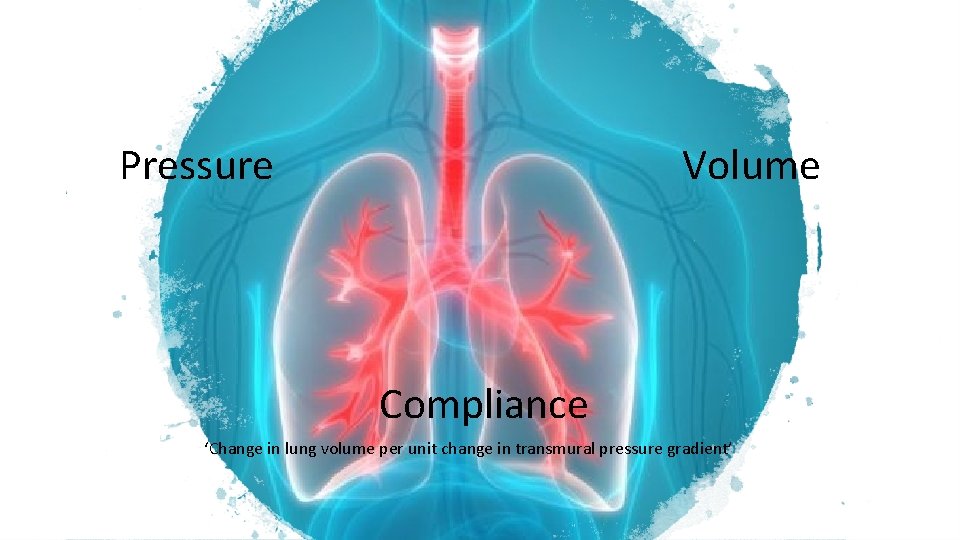
Pressure Volume Compliance ‘Change in lung volume per unit change in transmural pressure gradient’
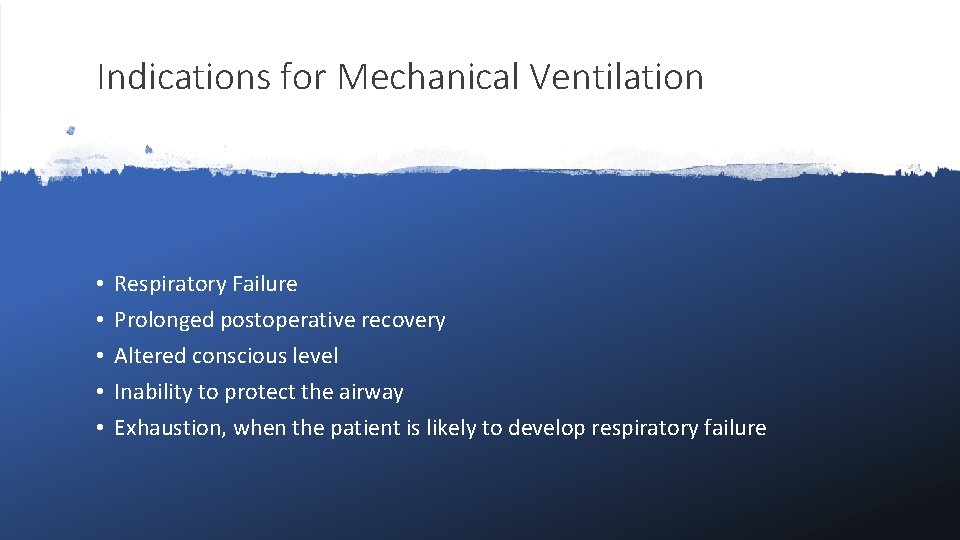
Indications for Mechanical Ventilation • • • Respiratory Failure Prolonged postoperative recovery Altered conscious level Inability to protect the airway Exhaustion, when the patient is likely to develop respiratory failure
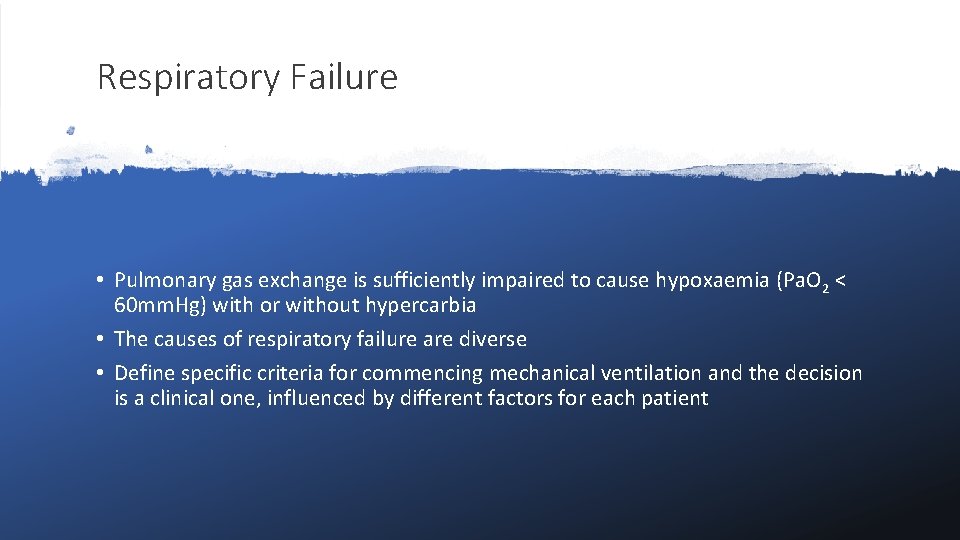
Respiratory Failure • Pulmonary gas exchange is sufficiently impaired to cause hypoxaemia (Pa. O 2 < 60 mm. Hg) with or without hypercarbia • The causes of respiratory failure are diverse • Define specific criteria for commencing mechanical ventilation and the decision is a clinical one, influenced by different factors for each patient
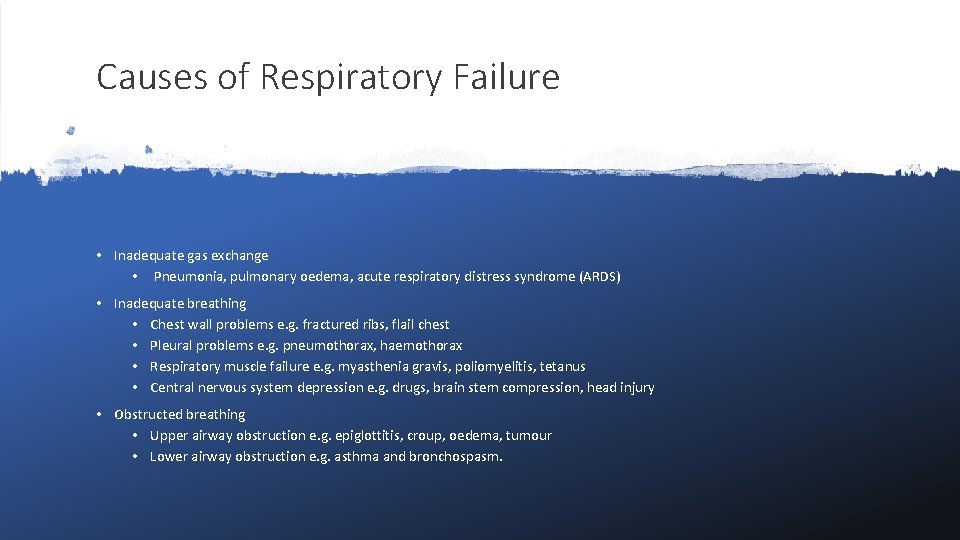
Causes of Respiratory Failure • Inadequate gas exchange • Pneumonia, pulmonary oedema, acute respiratory distress syndrome (ARDS) • Inadequate breathing • Chest wall problems e. g. fractured ribs, flail chest • Pleural problems e. g. pneumothorax, haemothorax • Respiratory muscle failure e. g. myasthenia gravis, poliomyelitis, tetanus • Central nervous system depression e. g. drugs, brain stem compression, head injury • Obstructed breathing • Upper airway obstruction e. g. epiglottitis, croup, oedema, tumour • Lower airway obstruction e. g. asthma and bronchospasm.
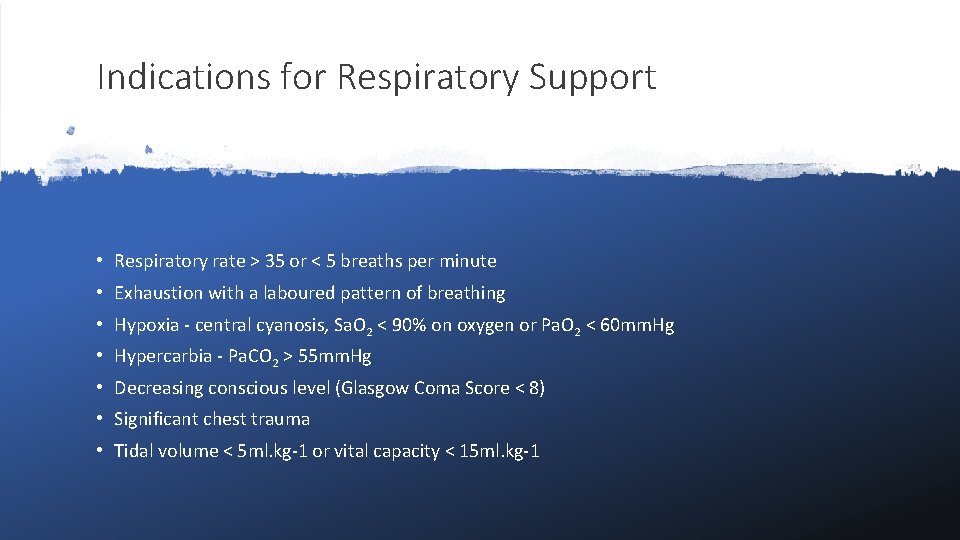
Indications for Respiratory Support • Respiratory rate > 35 or < 5 breaths per minute • Exhaustion with a laboured pattern of breathing • Hypoxia - central cyanosis, Sa. O 2 < 90% on oxygen or Pa. O 2 < 60 mm. Hg • Hypercarbia - Pa. CO 2 > 55 mm. Hg • Decreasing conscious level (Glasgow Coma Score < 8) • Significant chest trauma • Tidal volume < 5 ml. kg-1 or vital capacity < 15 ml. kg-1
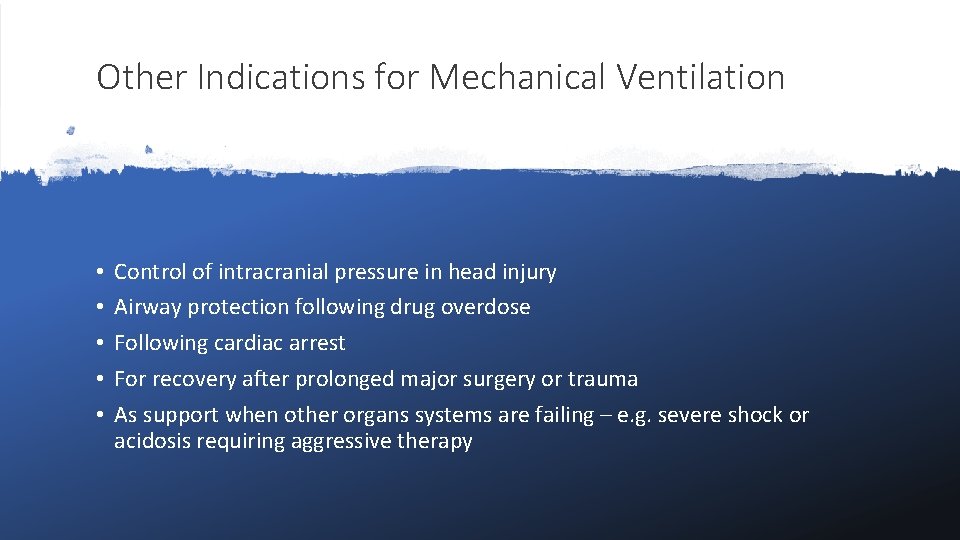
Other Indications for Mechanical Ventilation • • • Control of intracranial pressure in head injury Airway protection following drug overdose Following cardiac arrest For recovery after prolonged major surgery or trauma As support when other organs systems are failing – e. g. severe shock or acidosis requiring aggressive therapy
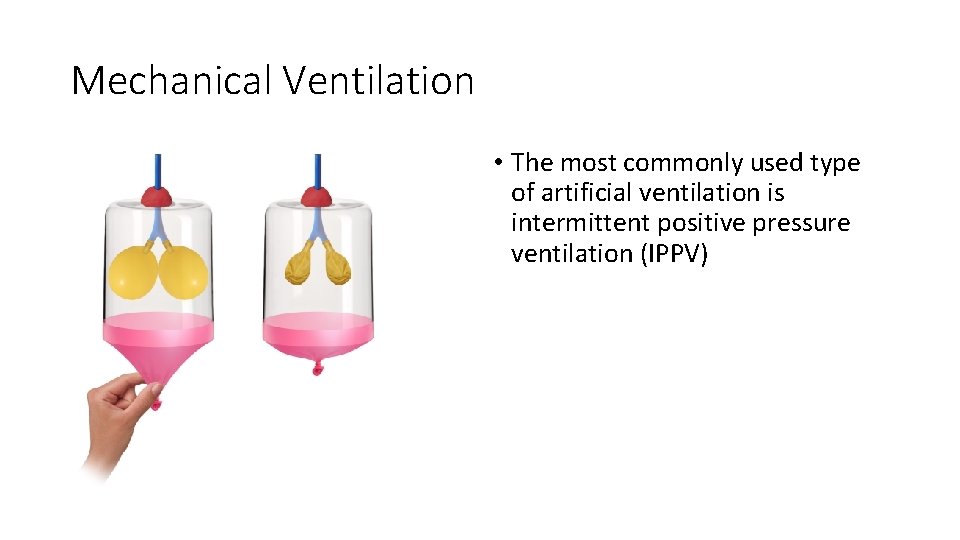
Mechanical Ventilation • The most commonly used type of artificial ventilation is intermittent positive pressure ventilation (IPPV)
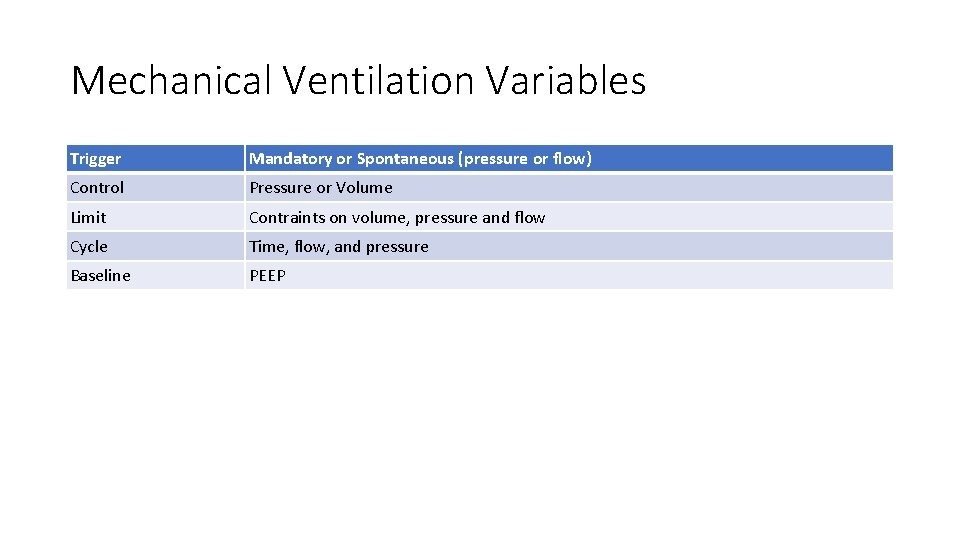
Mechanical Ventilation Variables Trigger Mandatory or Spontaneous (pressure or flow) Control Pressure or Volume Limit Contraints on volume, pressure and flow Cycle Time, flow, and pressure Baseline PEEP
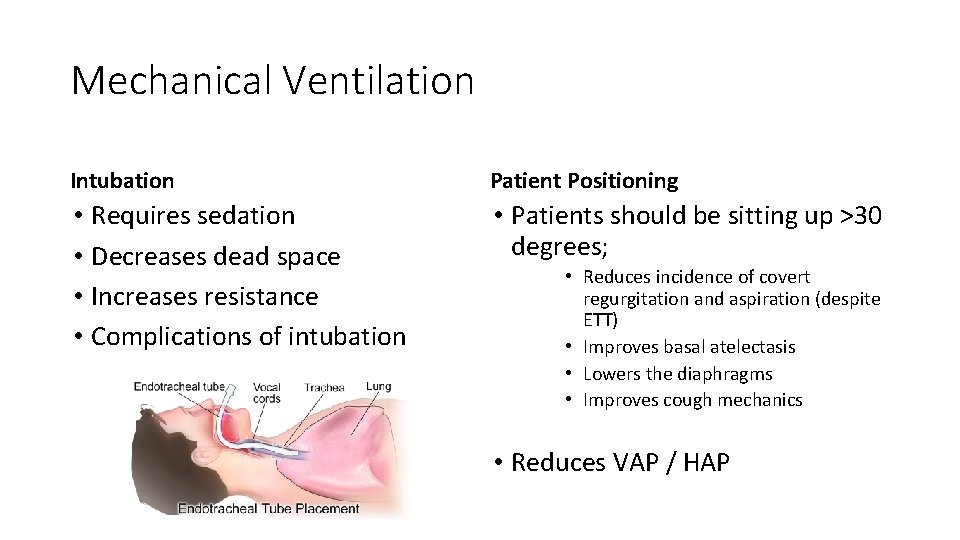
Mechanical Ventilation Intubation Patient Positioning • Requires sedation • Decreases dead space • Increases resistance • Complications of intubation • Patients should be sitting up >30 degrees; • Reduces incidence of covert regurgitation and aspiration (despite ETT) • Improves basal atelectasis • Lowers the diaphragms • Improves cough mechanics • Reduces VAP / HAP
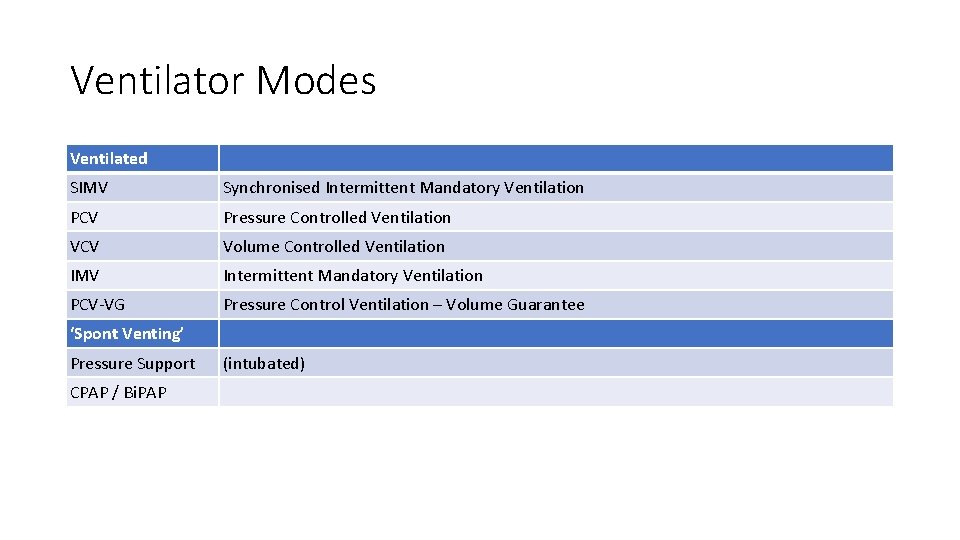
Ventilator Modes Ventilated SIMV Synchronised Intermittent Mandatory Ventilation PCV Pressure Controlled Ventilation VCV Volume Controlled Ventilation IMV Intermittent Mandatory Ventilation PCV-VG Pressure Control Ventilation – Volume Guarantee ‘Spont Venting’ Pressure Support CPAP / Bi. PAP (intubated)
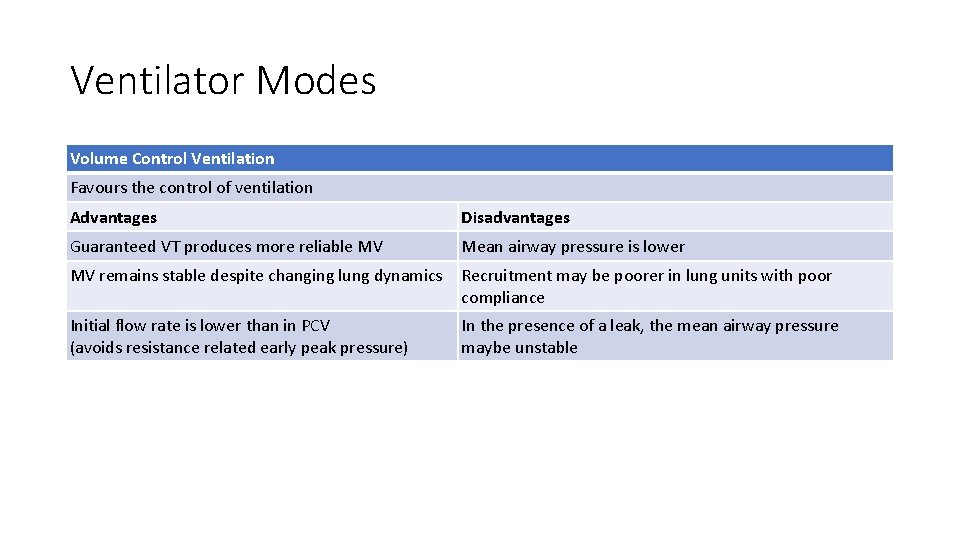
Ventilator Modes Volume Control Ventilation Favours the control of ventilation Advantages Disadvantages Guaranteed VT produces more reliable MV Mean airway pressure is lower MV remains stable despite changing lung dynamics Recruitment may be poorer in lung units with poor compliance Initial flow rate is lower than in PCV (avoids resistance related early peak pressure) In the presence of a leak, the mean airway pressure maybe unstable
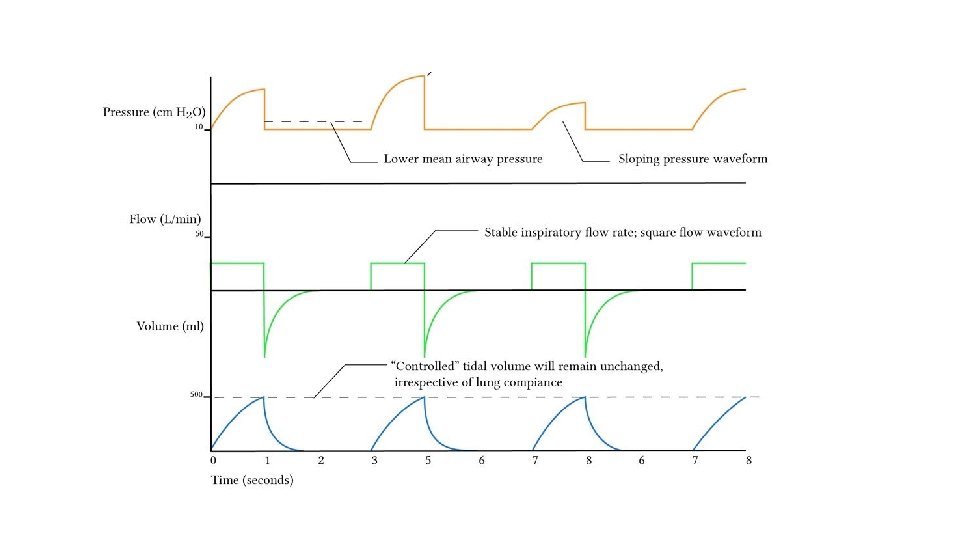
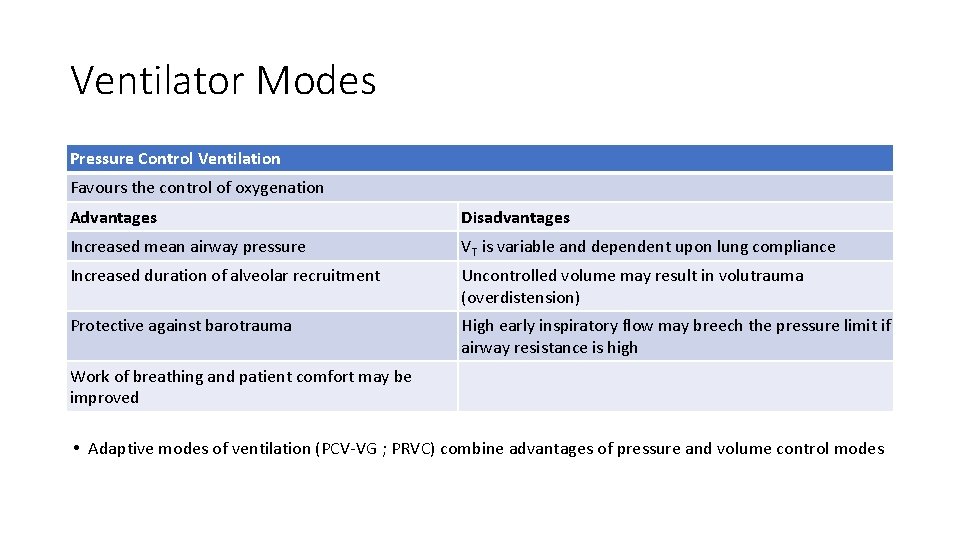
Ventilator Modes Pressure Control Ventilation Favours the control of oxygenation Advantages Disadvantages Increased mean airway pressure VT is variable and dependent upon lung compliance Increased duration of alveolar recruitment Uncontrolled volume may result in volutrauma (overdistension) Protective against barotrauma High early inspiratory flow may breech the pressure limit if airway resistance is high Work of breathing and patient comfort may be improved • Adaptive modes of ventilation (PCV-VG ; PRVC) combine advantages of pressure and volume control modes
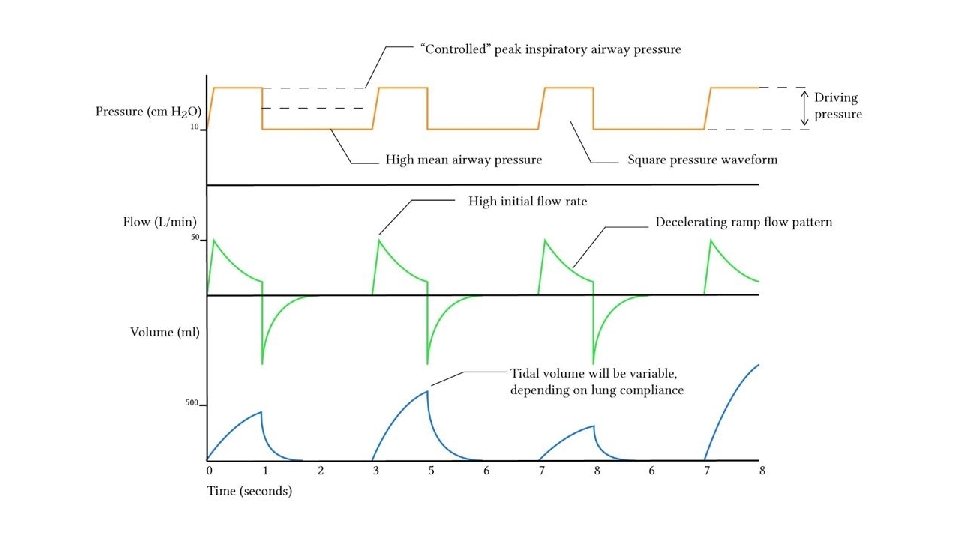
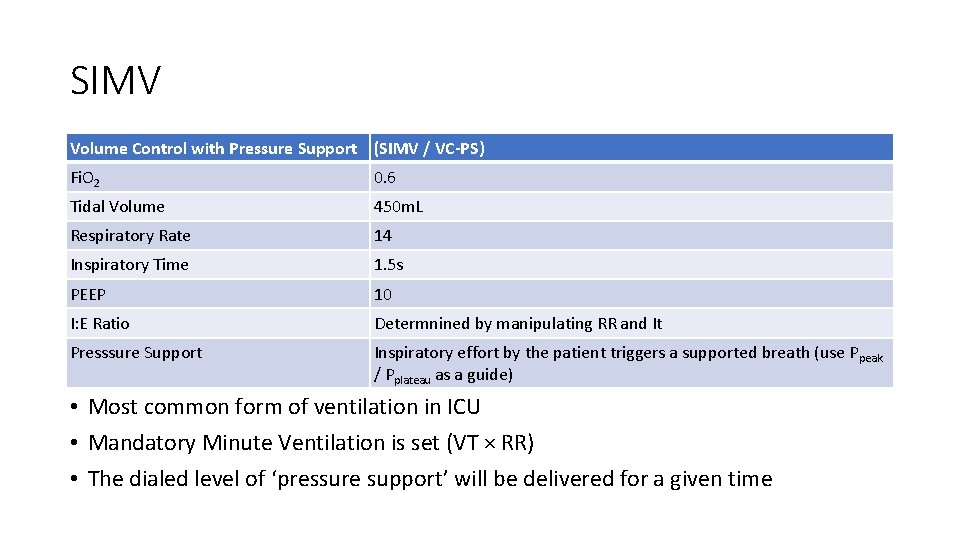
SIMV Volume Control with Pressure Support (SIMV / VC-PS) Fi. O 2 0. 6 Tidal Volume 450 m. L Respiratory Rate 14 Inspiratory Time 1. 5 s PEEP 10 I: E Ratio Determnined by manipulating RR and It Presssure Support Inspiratory effort by the patient triggers a supported breath (use Ppeak / Pplateau as a guide) • Most common form of ventilation in ICU • Mandatory Minute Ventilation is set (VT × RR) • The dialed level of ‘pressure support’ will be delivered for a given time
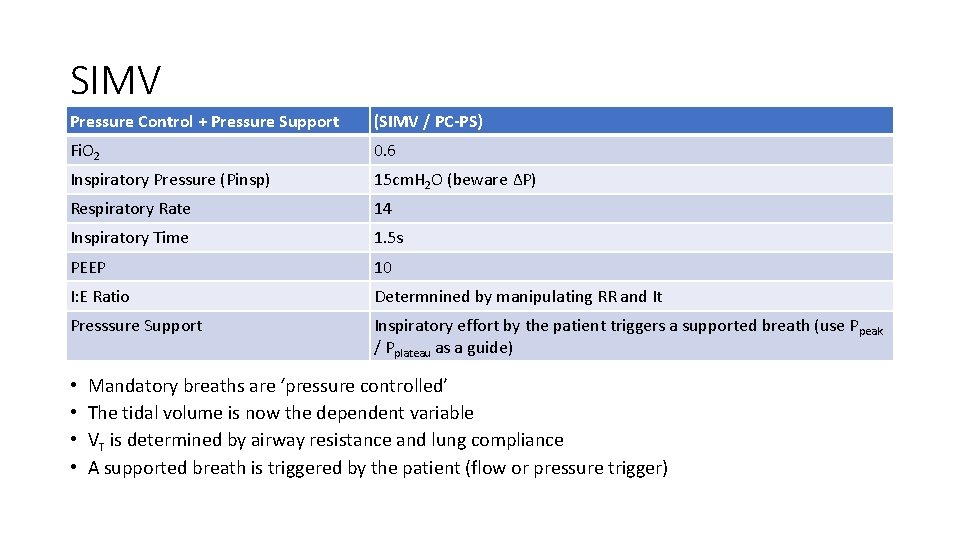
SIMV Pressure Control + Pressure Support (SIMV / PC-PS) Fi. O 2 0. 6 Inspiratory Pressure (Pinsp) 15 cm. H 2 O (beware ∆P) Respiratory Rate 14 Inspiratory Time 1. 5 s PEEP 10 I: E Ratio Determnined by manipulating RR and It Presssure Support Inspiratory effort by the patient triggers a supported breath (use Ppeak / Pplateau as a guide) • • Mandatory breaths are ‘pressure controlled’ The tidal volume is now the dependent variable VT is determined by airway resistance and lung compliance A supported breath is triggered by the patient (flow or pressure trigger)
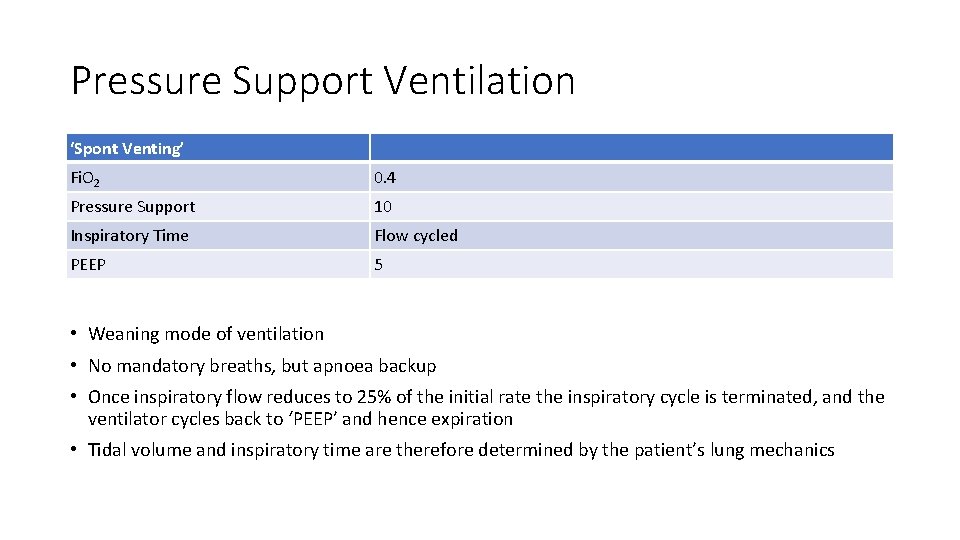
Pressure Support Ventilation ‘Spont Venting’ Fi. O 2 0. 4 Pressure Support 10 Inspiratory Time Flow cycled PEEP 5 • Weaning mode of ventilation • No mandatory breaths, but apnoea backup • Once inspiratory flow reduces to 25% of the initial rate the inspiratory cycle is terminated, and the ventilator cycles back to ‘PEEP’ and hence expiration • Tidal volume and inspiratory time are therefore determined by the patient’s lung mechanics
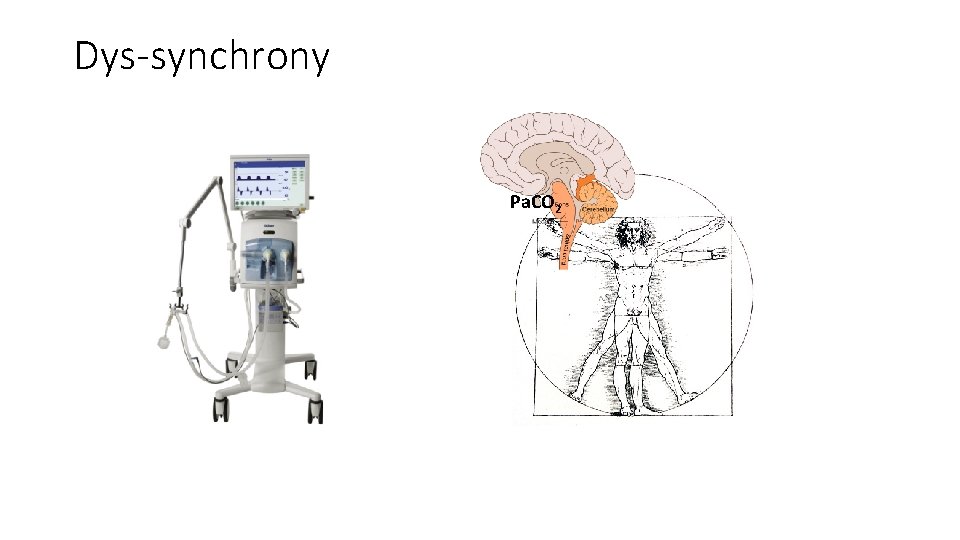
Dys-synchrony Pa. CO 2
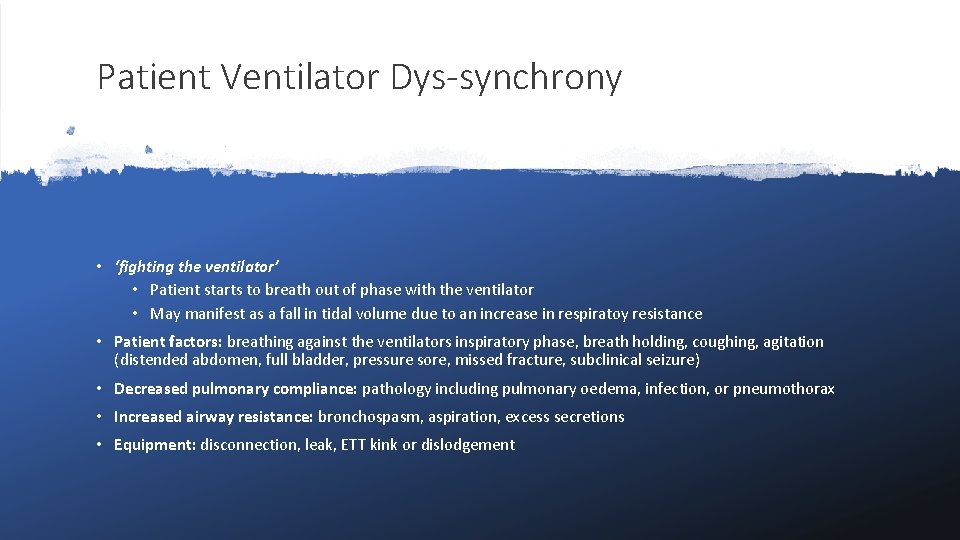
Patient Ventilator Dys-synchrony • ‘fighting the ventilator’ • Patient starts to breath out of phase with the ventilator • May manifest as a fall in tidal volume due to an increase in respiratoy resistance • Patient factors: breathing against the ventilators inspiratory phase, breath holding, coughing, agitation (distended abdomen, full bladder, pressure sore, missed fracture, subclinical seizure) • Decreased pulmonary compliance: pathology including pulmonary oedema, infection, or pneumothorax • Increased airway resistance: bronchospasm, aspiration, excess secretions • Equipment: disconnection, leak, ETT kink or dislodgement
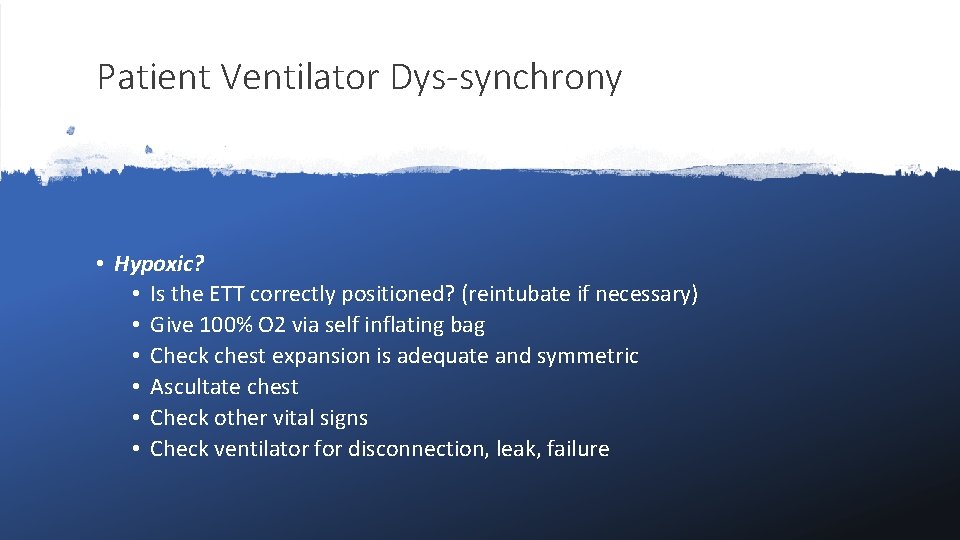
Patient Ventilator Dys-synchrony • Hypoxic? • Is the ETT correctly positioned? (reintubate if necessary) • Give 100% O 2 via self inflating bag • Check chest expansion is adequate and symmetric • Ascultate chest • Check other vital signs • Check ventilator for disconnection, leak, failure
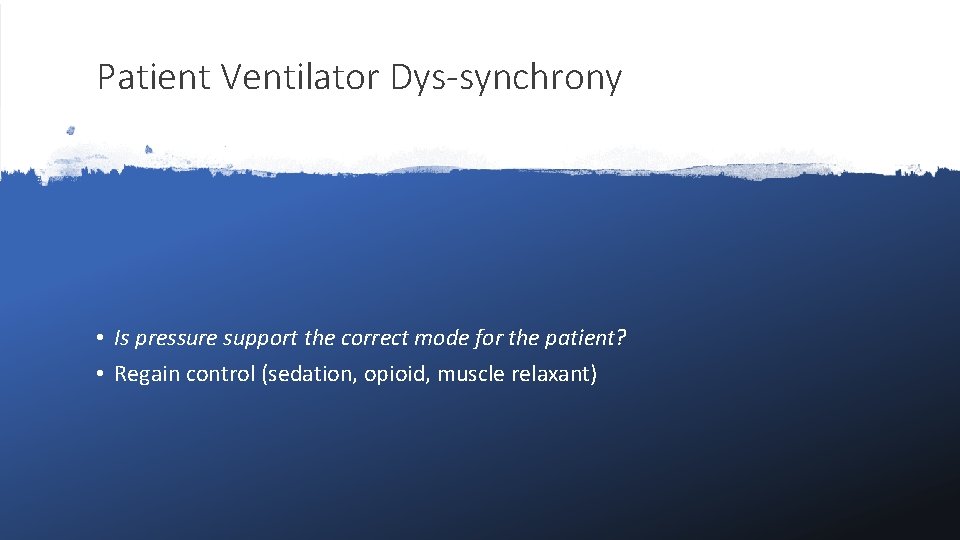
Patient Ventilator Dys-synchrony • Is pressure support the correct mode for the patient? • Regain control (sedation, opioid, muscle relaxant)
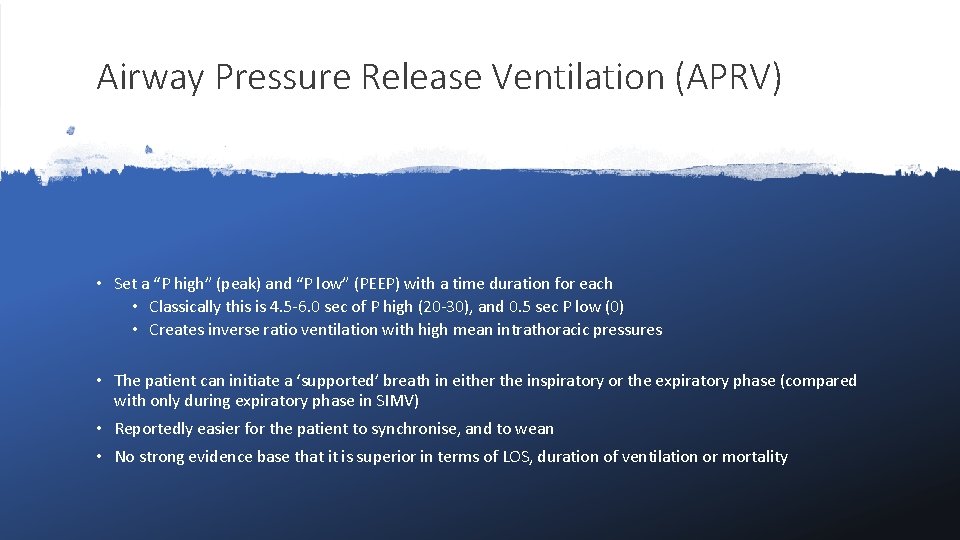
Airway Pressure Release Ventilation (APRV) • Set a “P high” (peak) and “P low” (PEEP) with a time duration for each • Classically this is 4. 5 -6. 0 sec of P high (20 -30), and 0. 5 sec P low (0) • Creates inverse ratio ventilation with high mean intrathoracic pressures • The patient can initiate a ‘supported’ breath in either the inspiratory or the expiratory phase (compared with only during expiratory phase in SIMV) • Reportedly easier for the patient to synchronise, and to wean • No strong evidence base that it is superior in terms of LOS, duration of ventilation or mortality
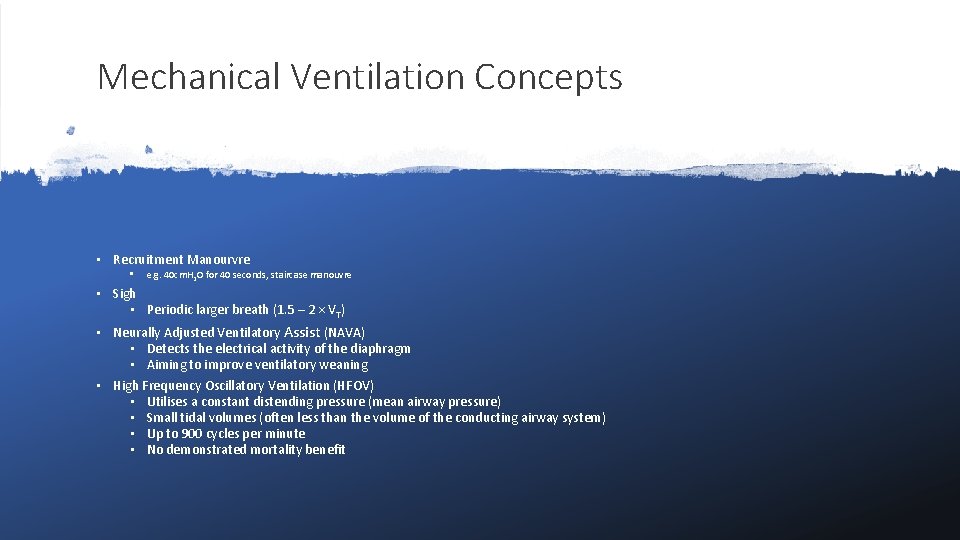
Mechanical Ventilation Concepts • Recruitment Manourvre • e. g. 40 cm. H 2 O for 40 seconds, staircase manouvre • Sigh • Periodic larger breath (1. 5 – 2 × VT) • Neurally Adjusted Ventilatory Assist (NAVA) • Detects the electrical activity of the diaphragm • Aiming to improve ventilatory weaning • High Frequency Oscillatory Ventilation (HFOV) • Utilises a constant distending pressure (mean airway pressure) • Small tidal volumes (often less than the volume of the conducting airway system) • Up to 900 cycles per minute • No demonstrated mortality benefit
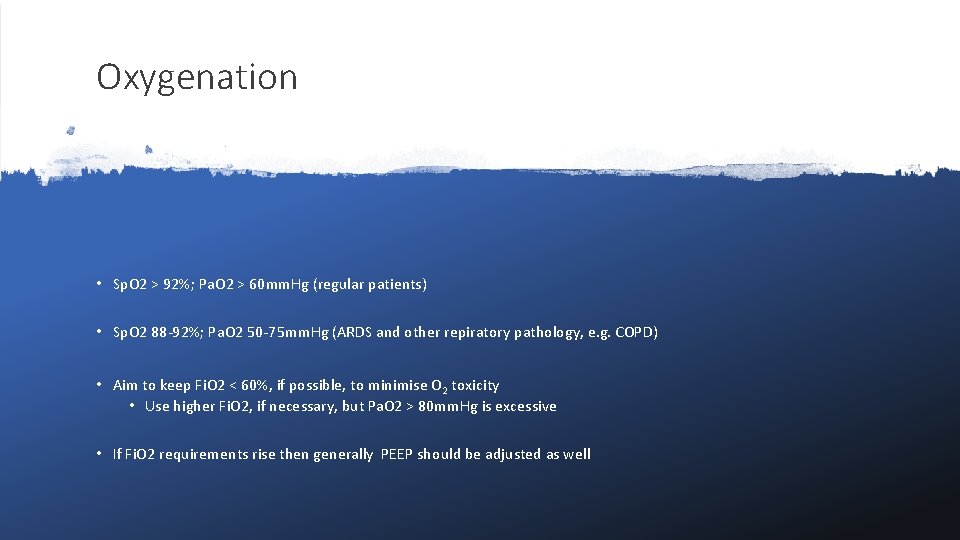
Oxygenation • Sp. O 2 > 92%; Pa. O 2 > 60 mm. Hg (regular patients) • Sp. O 2 88 -92%; Pa. O 2 50 -75 mm. Hg (ARDS and other repiratory pathology, e. g. COPD) • Aim to keep Fi. O 2 < 60%, if possible, to minimise O 2 toxicity • Use higher Fi. O 2, if necessary, but Pa. O 2 > 80 mm. Hg is excessive • If Fi. O 2 requirements rise then generally PEEP should be adjusted as well
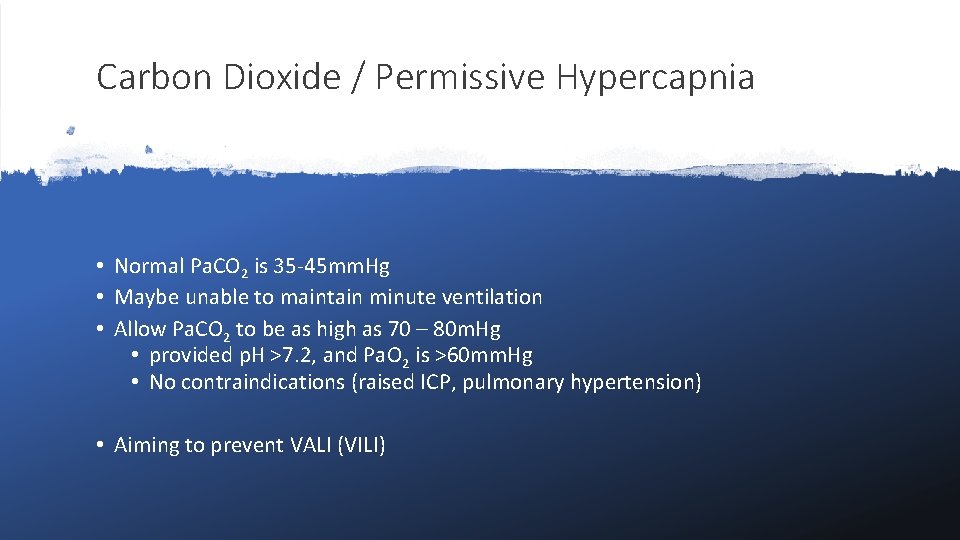
Carbon Dioxide / Permissive Hypercapnia • Normal Pa. CO 2 is 35 -45 mm. Hg • Maybe unable to maintain minute ventilation • Allow Pa. CO 2 to be as high as 70 – 80 m. Hg • provided p. H >7. 2, and Pa. O 2 is >60 mm. Hg • No contraindications (raised ICP, pulmonary hypertension) • Aiming to prevent VALI (VILI)
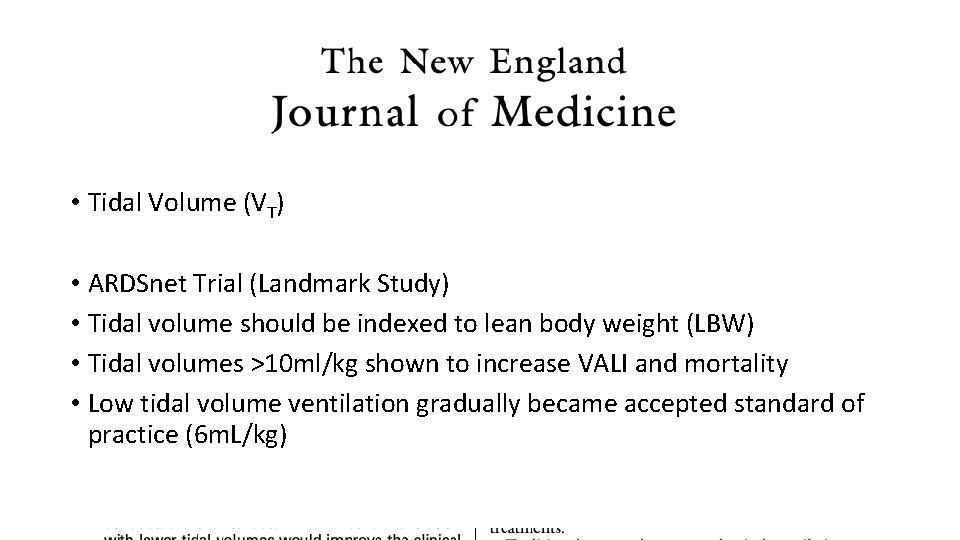
• Tidal Volume (VT) • ARDSnet Trial (Landmark Study) • Tidal volume should be indexed to lean body weight (LBW) • Tidal volumes >10 ml/kg shown to increase VALI and mortality • Low tidal volume ventilation gradually became accepted standard of practice (6 m. L/kg)
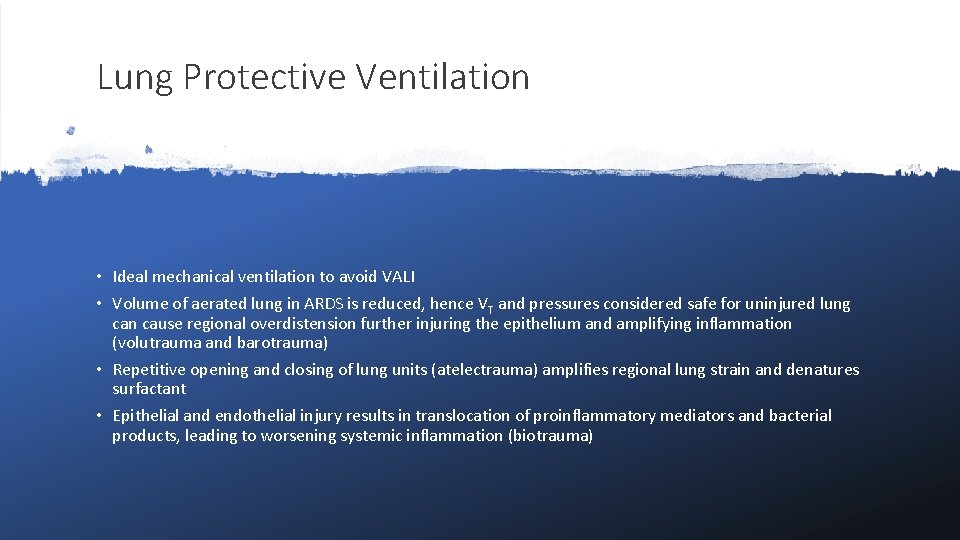
Lung Protective Ventilation • Ideal mechanical ventilation to avoid VALI • Volume of aerated lung in ARDS is reduced, hence VT and pressures considered safe for uninjured lung can cause regional overdistension further injuring the epithelium and amplifying inflammation (volutrauma and barotrauma) • Repetitive opening and closing of lung units (atelectrauma) amplifies regional lung strain and denatures surfactant • Epithelial and endothelial injury results in translocation of proinflammatory mediators and bacterial products, leading to worsening systemic inflammation (biotrauma)
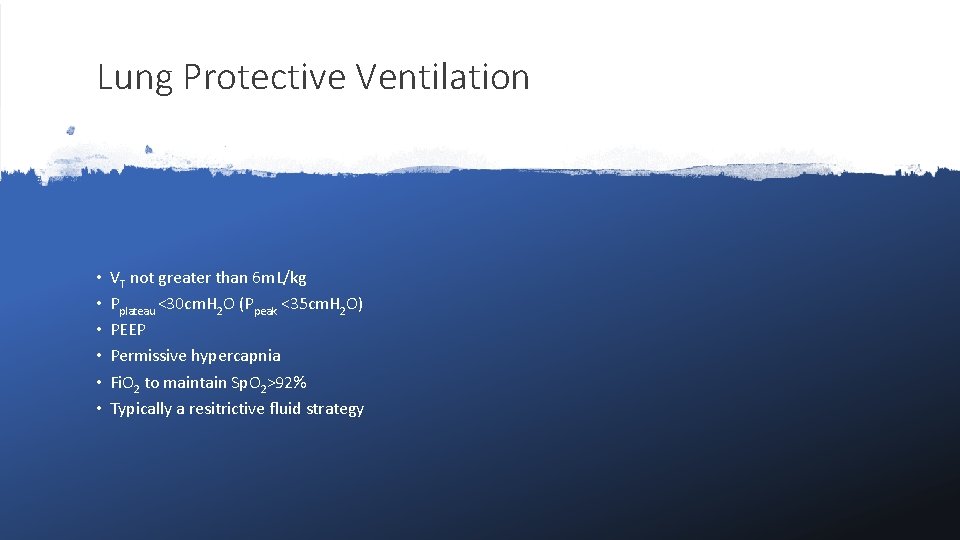
Lung Protective Ventilation • • • VT not greater than 6 m. L/kg Pplateau <30 cm. H 2 O (Ppeak <35 cm. H 2 O) PEEP Permissive hypercapnia Fi. O 2 to maintain Sp. O 2>92% Typically a resitrictive fluid strategy
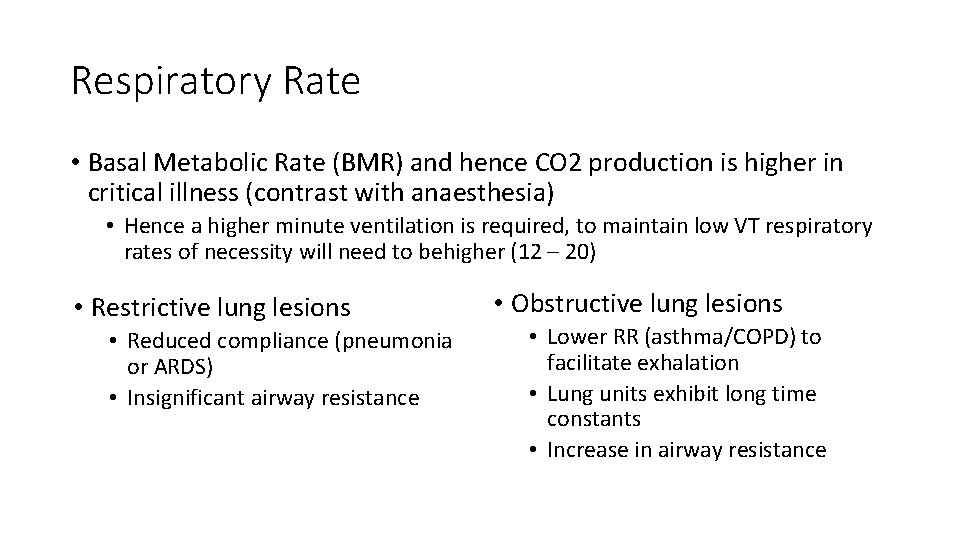
Respiratory Rate • Basal Metabolic Rate (BMR) and hence CO 2 production is higher in critical illness (contrast with anaesthesia) • Hence a higher minute ventilation is required, to maintain low VT respiratory rates of necessity will need to behigher (12 – 20) • Restrictive lung lesions • Reduced compliance (pneumonia or ARDS) • Insignificant airway resistance • Obstructive lung lesions • Lower RR (asthma/COPD) to facilitate exhalation • Lung units exhibit long time constants • Increase in airway resistance
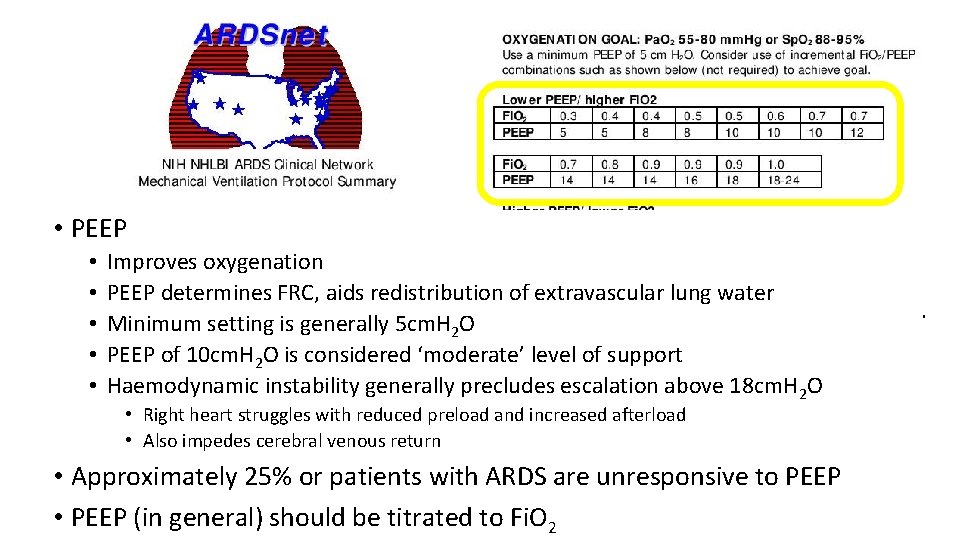
• PEEP • • • Improves oxygenation PEEP determines FRC, aids redistribution of extravascular lung water Minimum setting is generally 5 cm. H 2 O PEEP of 10 cm. H 2 O is considered ‘moderate’ level of support Haemodynamic instability generally precludes escalation above 18 cm. H 2 O • Right heart struggles with reduced preload and increased afterload • Also impedes cerebral venous return • Approximately 25% or patients with ARDS are unresponsive to PEEP • PEEP (in general) should be titrated to Fi. O 2
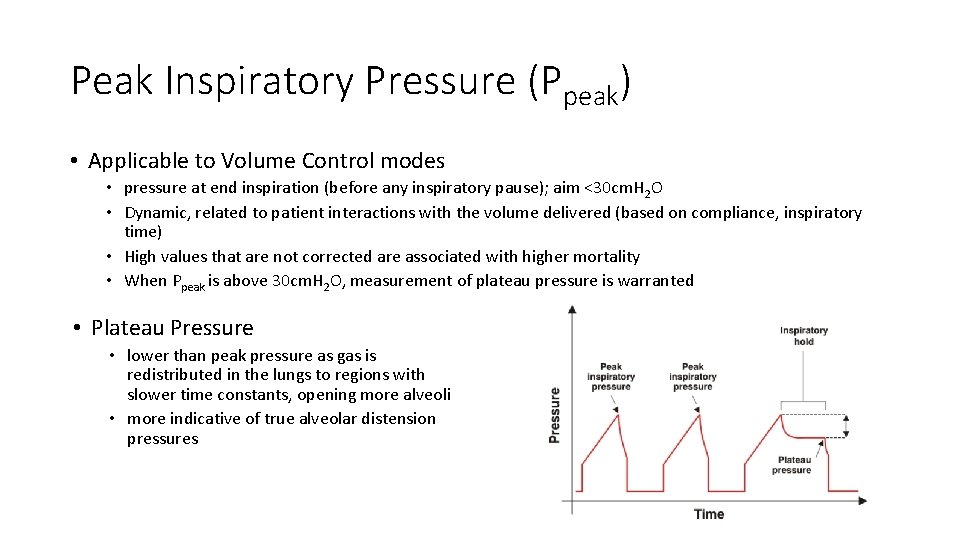
Peak Inspiratory Pressure (Ppeak) • Applicable to Volume Control modes • pressure at end inspiration (before any inspiratory pause); aim <30 cm. H 2 O • Dynamic, related to patient interactions with the volume delivered (based on compliance, inspiratory time) • High values that are not corrected are associated with higher mortality • When Ppeak is above 30 cm. H 2 O, measurement of plateau pressure is warranted • Plateau Pressure • lower than peak pressure as gas is redistributed in the lungs to regions with slower time constants, opening more alveoli • more indicative of true alveolar distension pressures
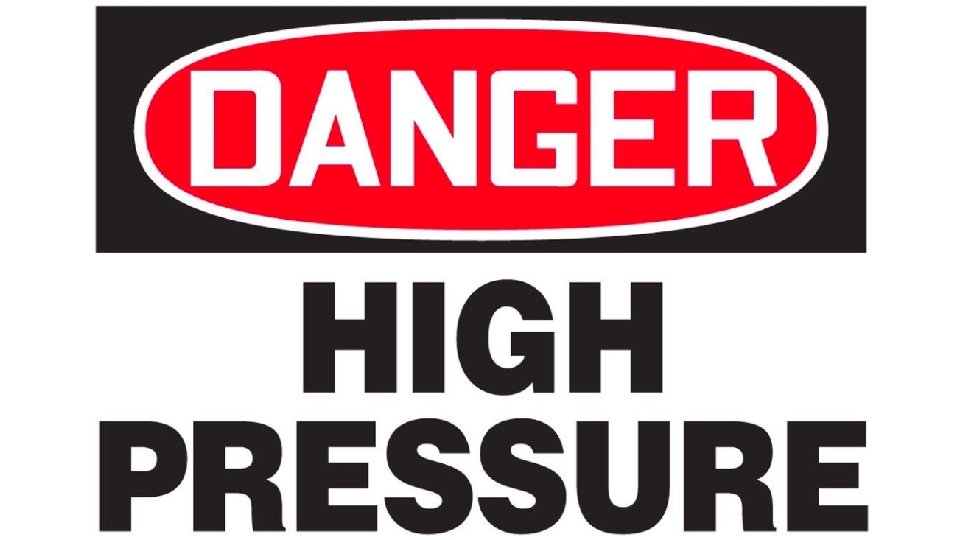
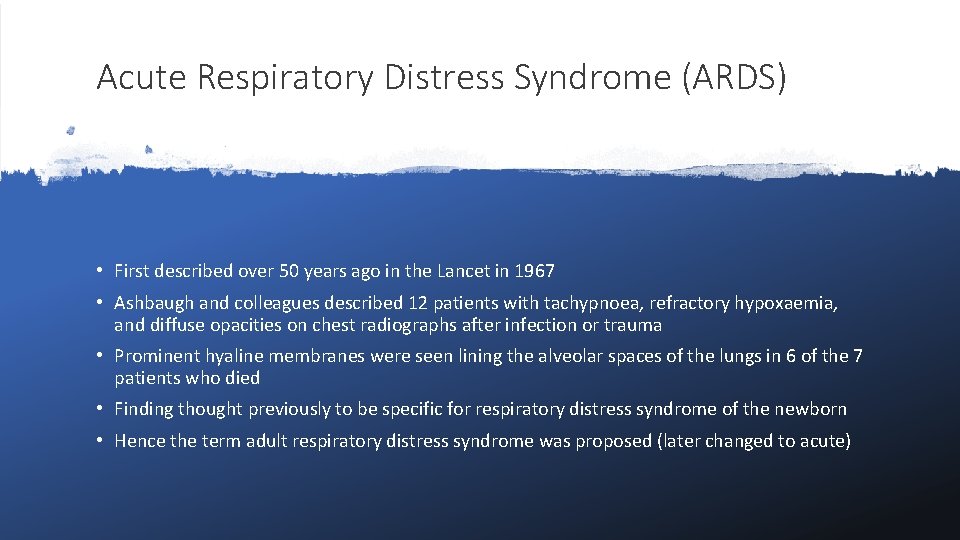
Acute Respiratory Distress Syndrome (ARDS) • First described over 50 years ago in the Lancet in 1967 • Ashbaugh and colleagues described 12 patients with tachypnoea, refractory hypoxaemia, and diffuse opacities on chest radiographs after infection or trauma • Prominent hyaline membranes were seen lining the alveolar spaces of the lungs in 6 of the 7 patients who died • Finding thought previously to be specific for respiratory distress syndrome of the newborn • Hence the term adult respiratory distress syndrome was proposed (later changed to acute)
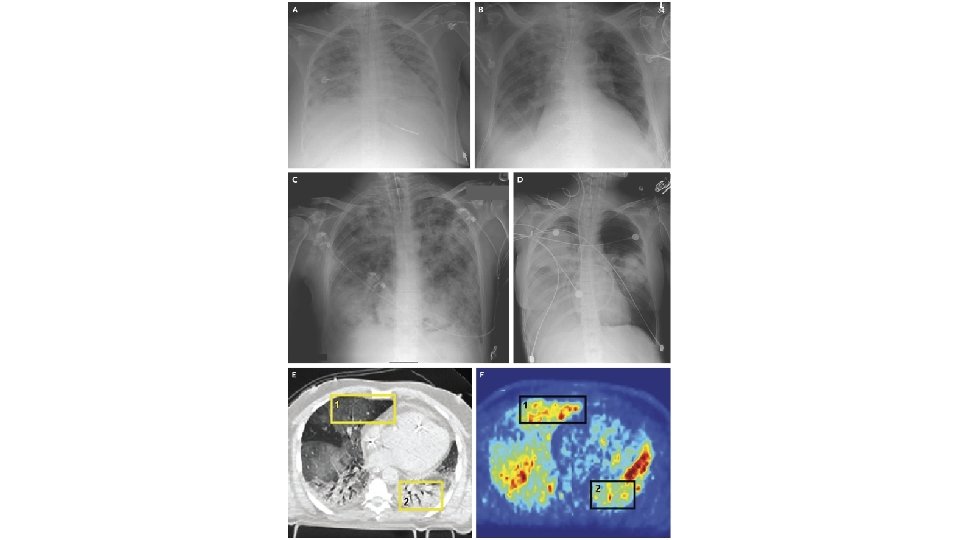
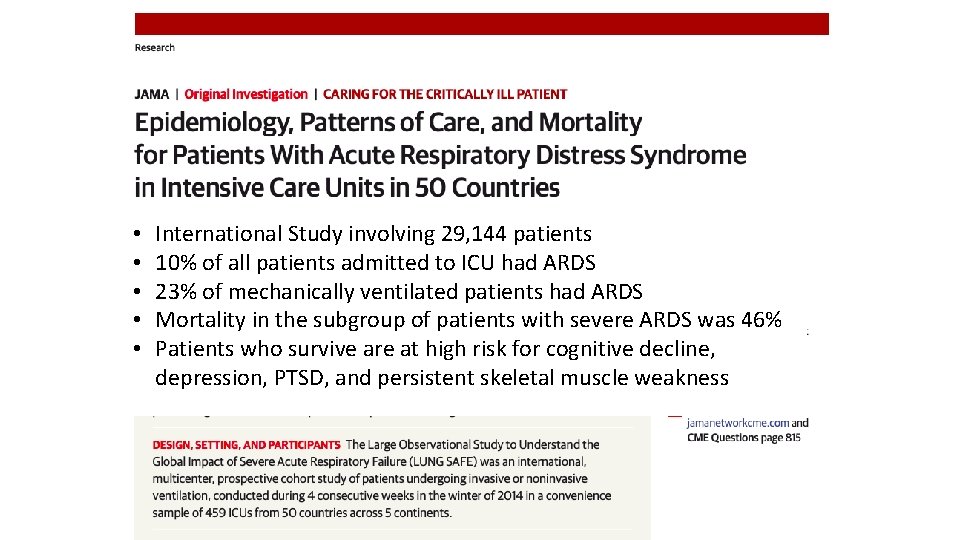
• • • International Study involving 29, 144 patients 10% of all patients admitted to ICU had ARDS 23% of mechanically ventilated patients had ARDS Mortality in the subgroup of patients with severe ARDS was 46% Patients who survive are at high risk for cognitive decline, depression, PTSD, and persistent skeletal muscle weakness
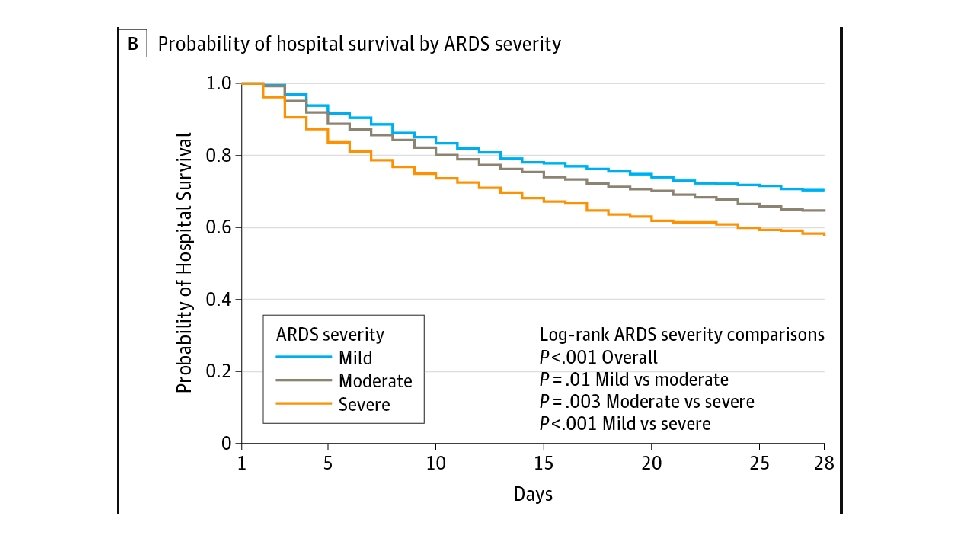
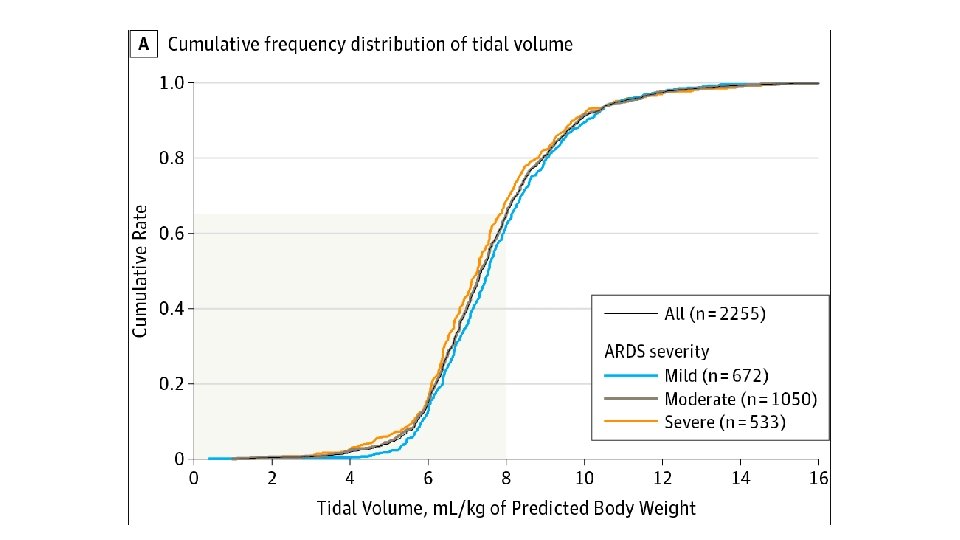
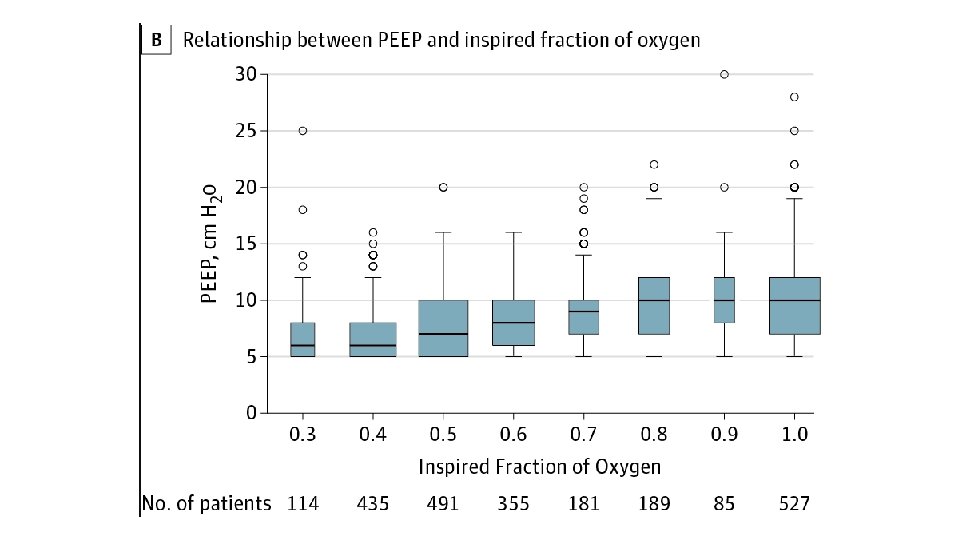
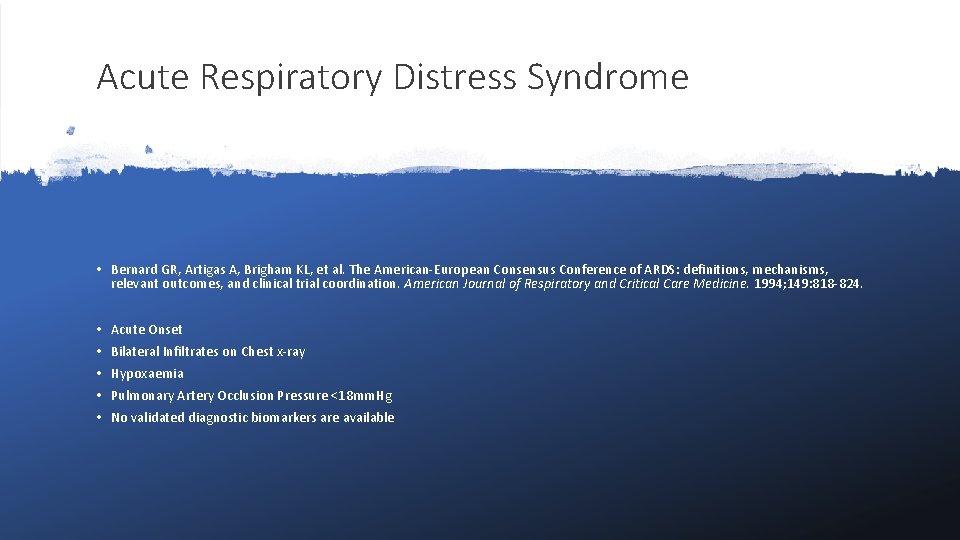
Acute Respiratory Distress Syndrome • Bernard GR, Artigas A, Brigham KL, et al. The American-European Consensus Conference of ARDS: definitions, mechanisms, relevant outcomes, and clinical trial coordination. American Journal of Respiratory and Critical Care Medicine. 1994; 149: 818 -824. • • • Acute Onset Bilateral Infiltrates on Chest x-ray Hypoxaemia Pulmonary Artery Occlusion Pressure <18 mm. Hg No validated diagnostic biomarkers are available
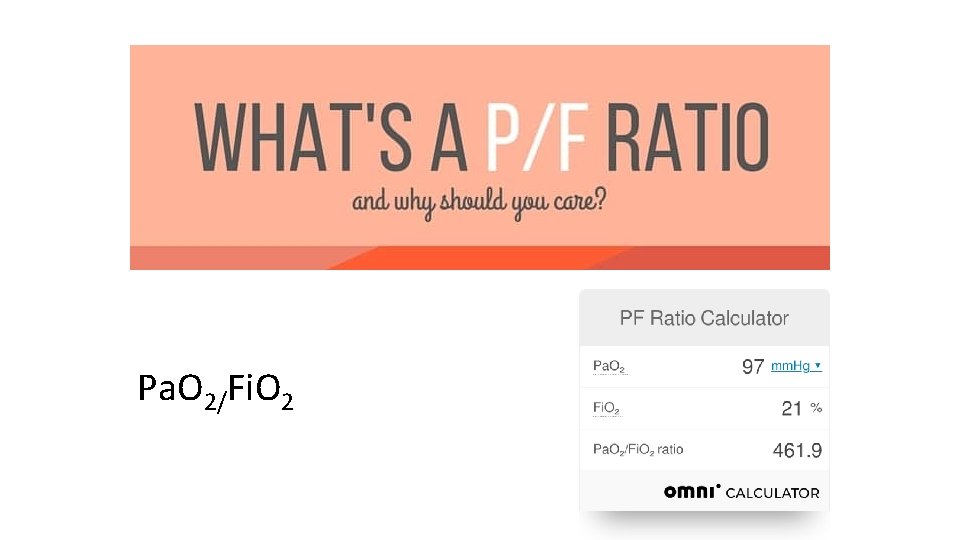
Pa. O 2/Fi. O 2
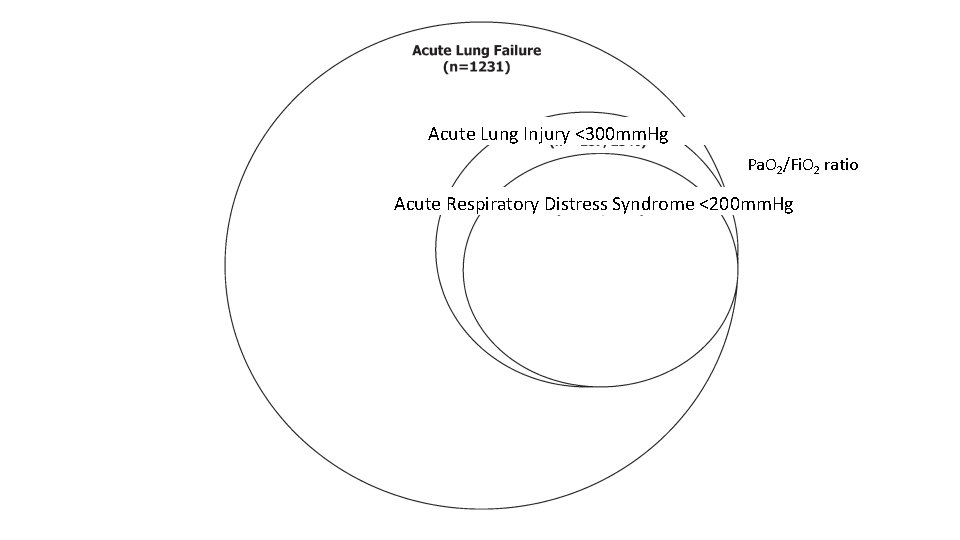
Acute Lung Injury <300 mm. Hg Pa. O 2/Fi. O 2 ratio Acute Respiratory Distress Syndrome <200 mm. Hg
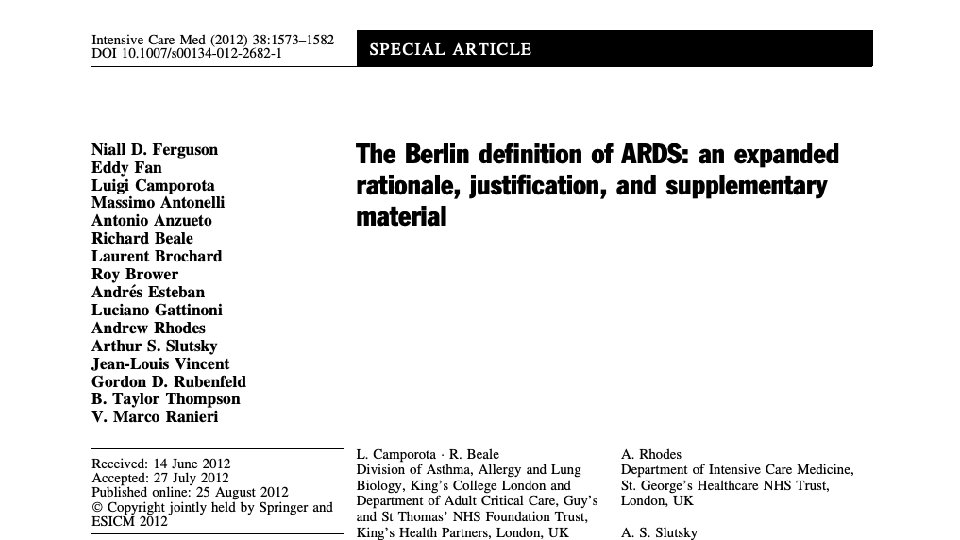
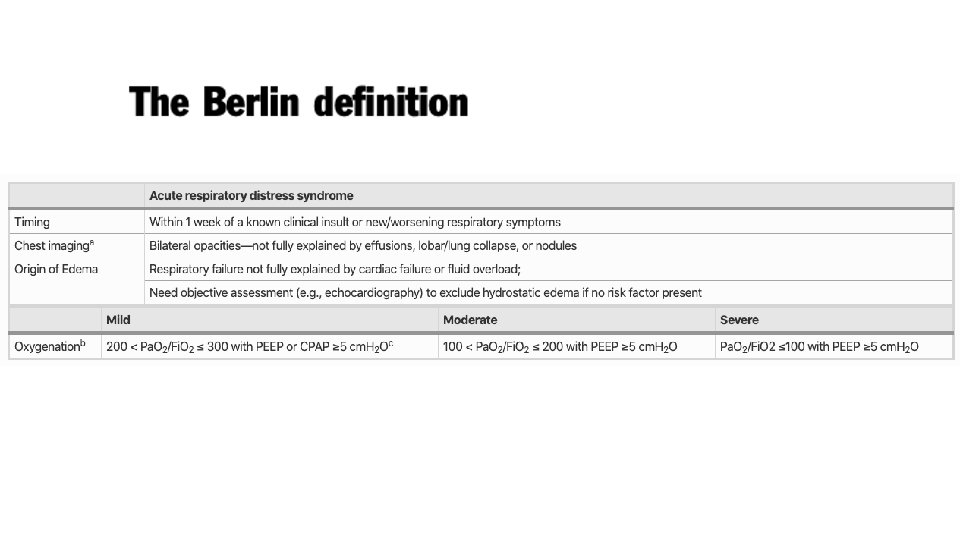
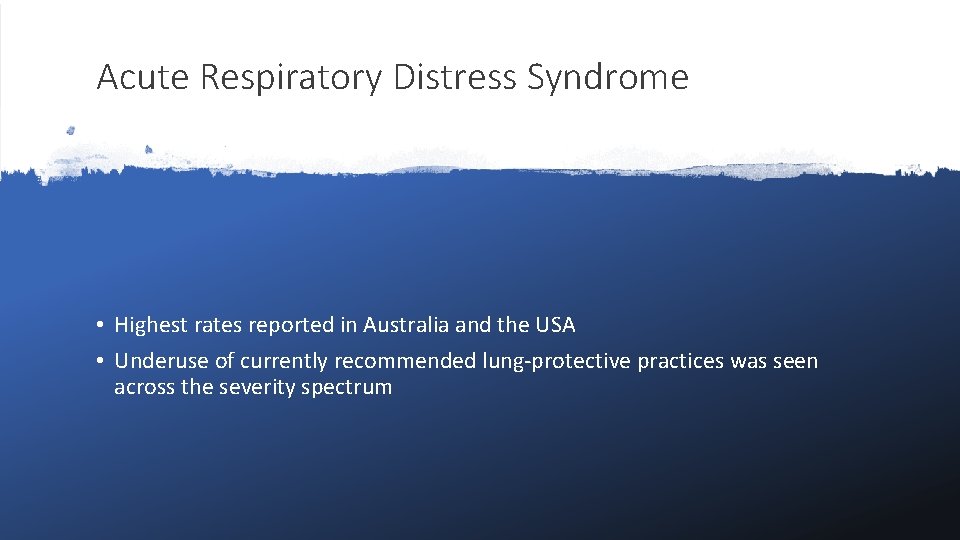
Acute Respiratory Distress Syndrome • Highest rates reported in Australia and the USA • Underuse of currently recommended lung-protective practices was seen across the severity spectrum
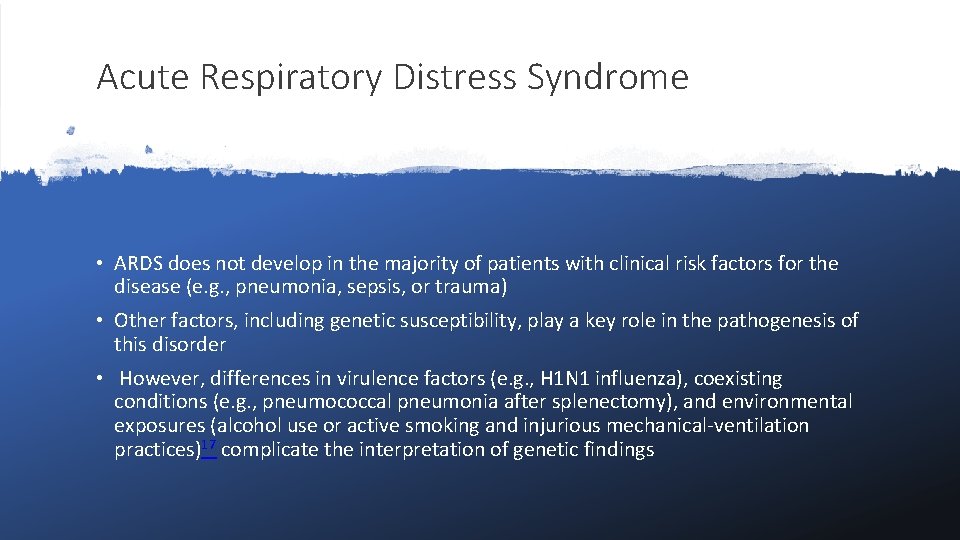
Acute Respiratory Distress Syndrome • ARDS does not develop in the majority of patients with clinical risk factors for the disease (e. g. , pneumonia, sepsis, or trauma) • Other factors, including genetic susceptibility, play a key role in the pathogenesis of this disorder • However, differences in virulence factors (e. g. , H 1 N 1 influenza), coexisting conditions (e. g. , pneumococcal pneumonia after splenectomy), and environmental exposures (alcohol use or active smoking and injurious mechanical-ventilation practices)17 complicate the interpretation of genetic findings
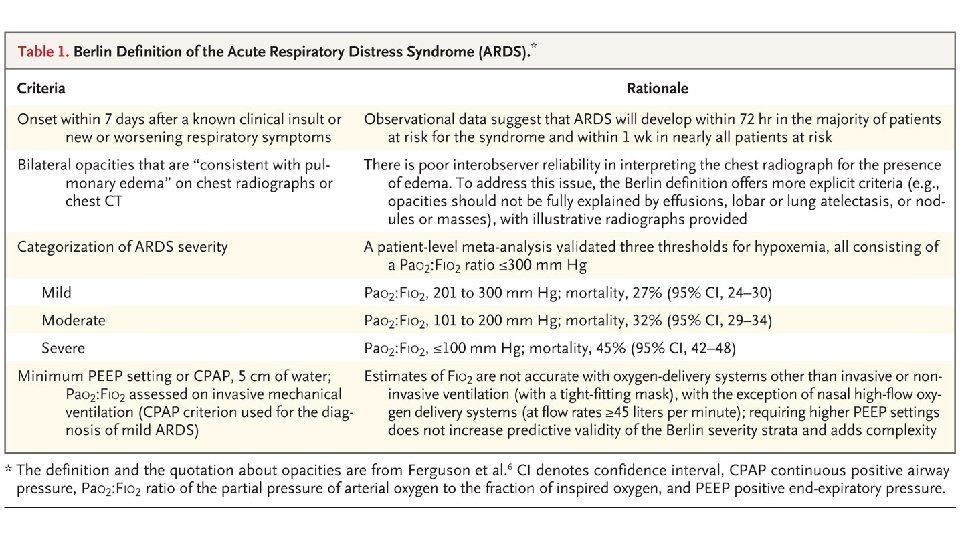
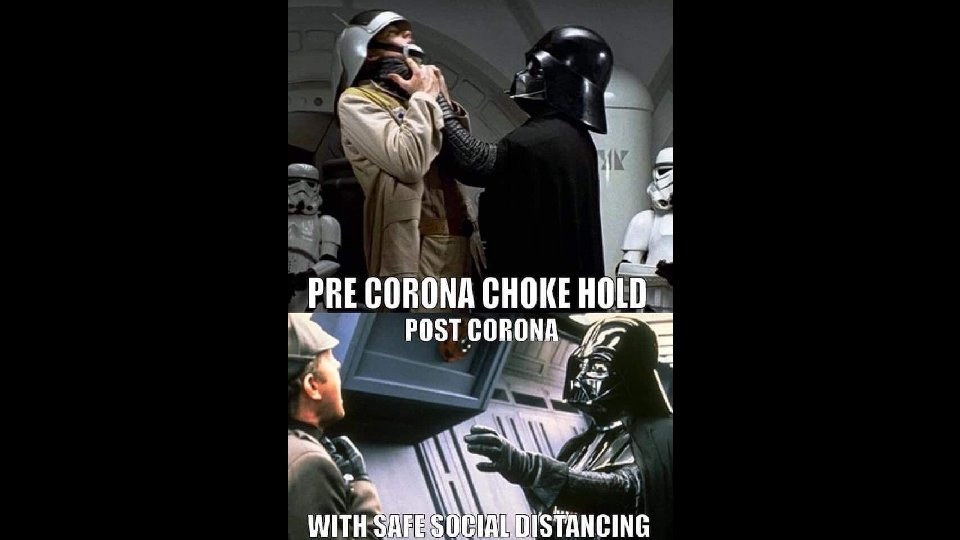
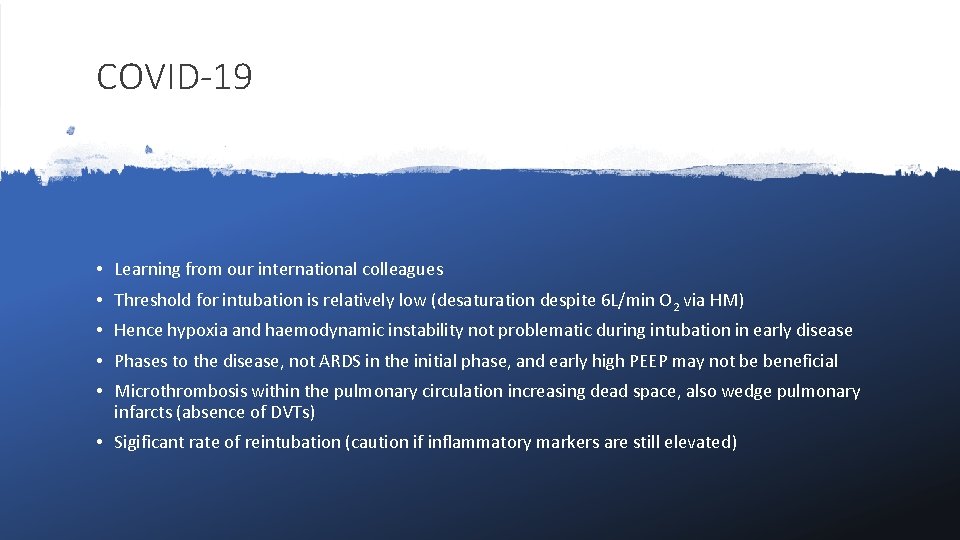
COVID-19 • Learning from our international colleagues • Threshold for intubation is relatively low (desaturation despite 6 L/min O 2 via HM) • Hence hypoxia and haemodynamic instability not problematic during intubation in early disease • Phases to the disease, not ARDS in the initial phase, and early high PEEP may not be beneficial • Microthrombosis within the pulmonary circulation increasing dead space, also wedge pulmonary infarcts (absence of DVTs) • Sigificant rate of reintubation (caution if inflammatory markers are still elevated)
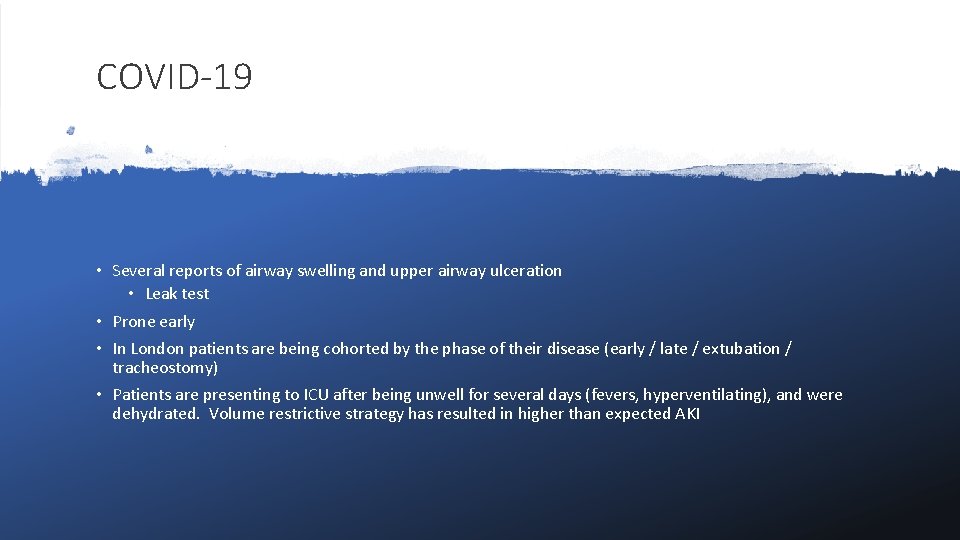
COVID-19 • Several reports of airway swelling and upper airway ulceration • Leak test • Prone early • In London patients are being cohorted by the phase of their disease (early / late / extubation / tracheostomy) • Patients are presenting to ICU after being unwell for several days (fevers, hyperventilating), and were dehydrated. Volume restrictive strategy has resulted in higher than expected AKI