Lecture Presentation Chapter 19 Radioactivity and Nuclear Chemistry
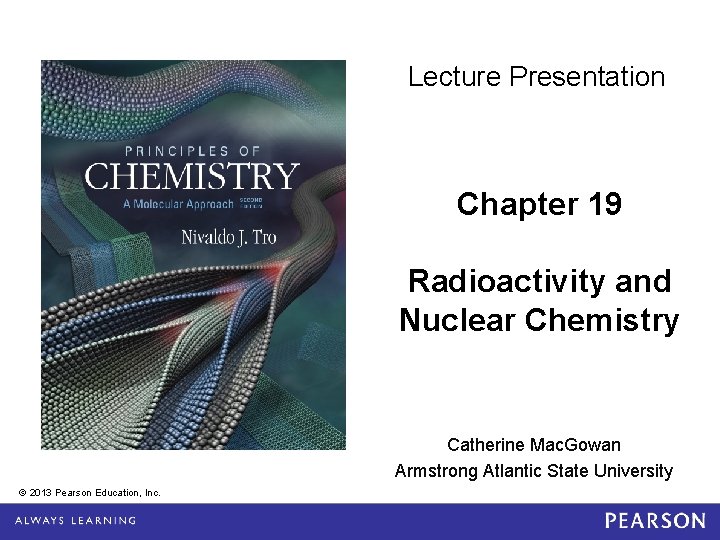
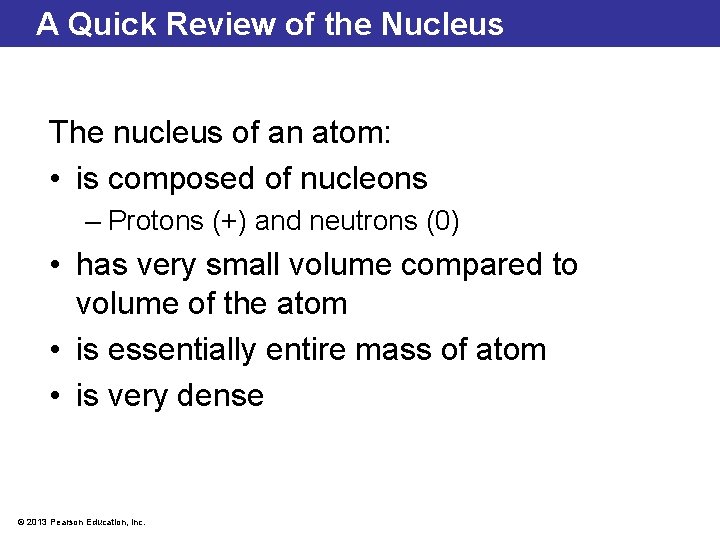
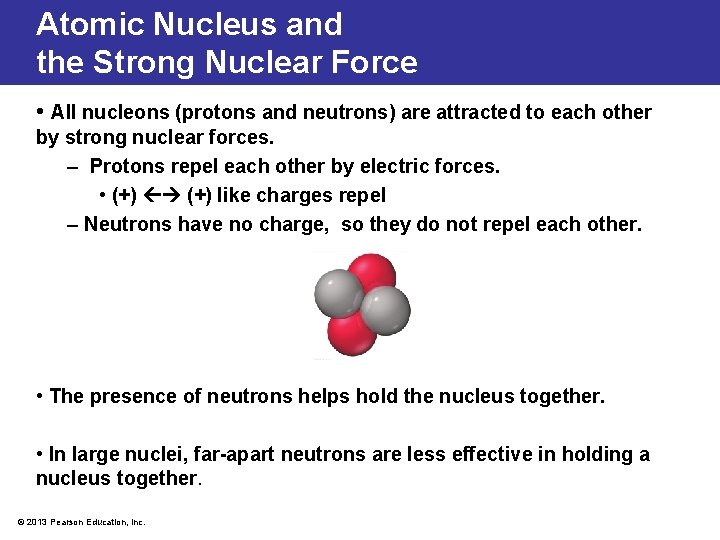
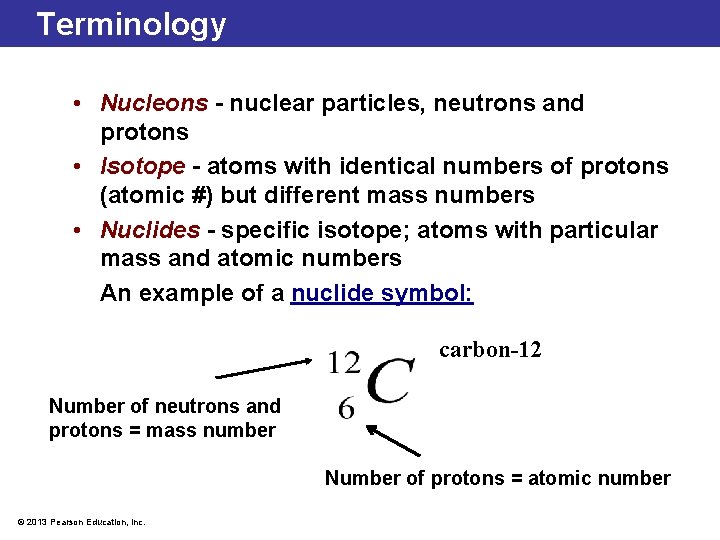
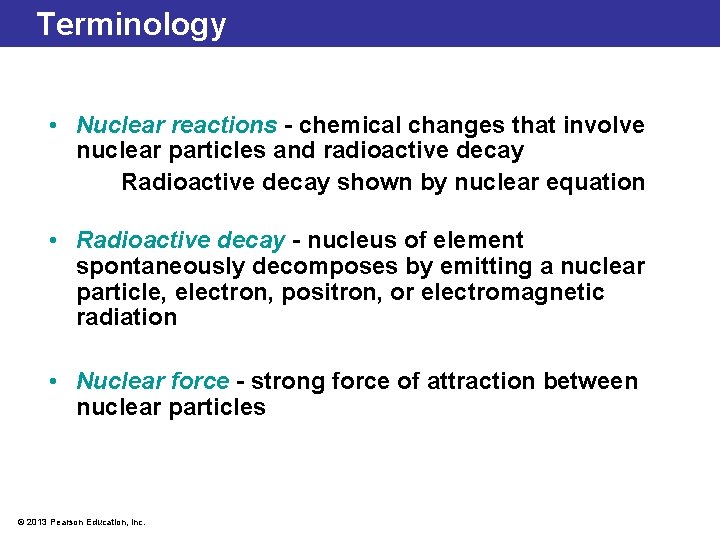
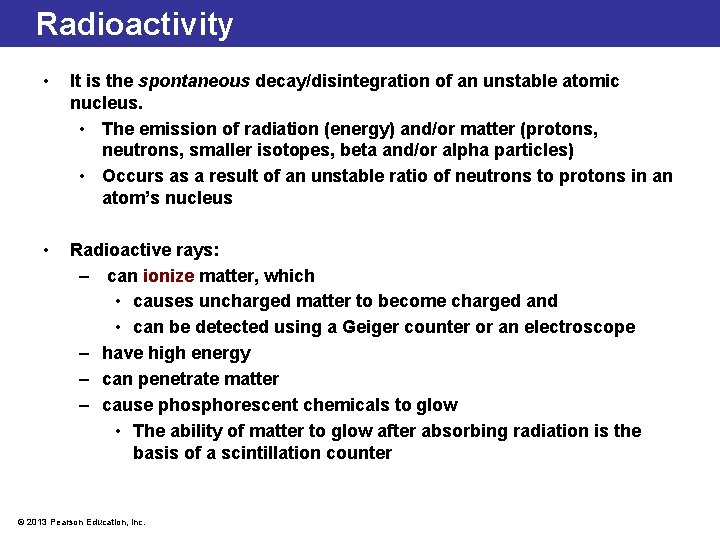
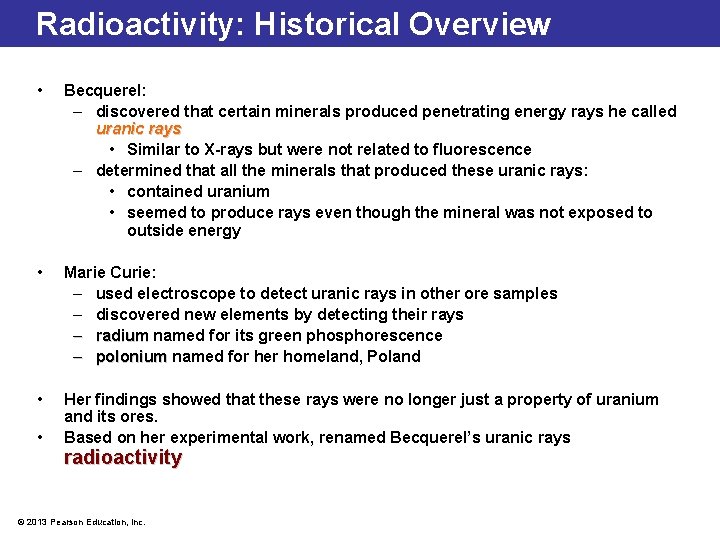
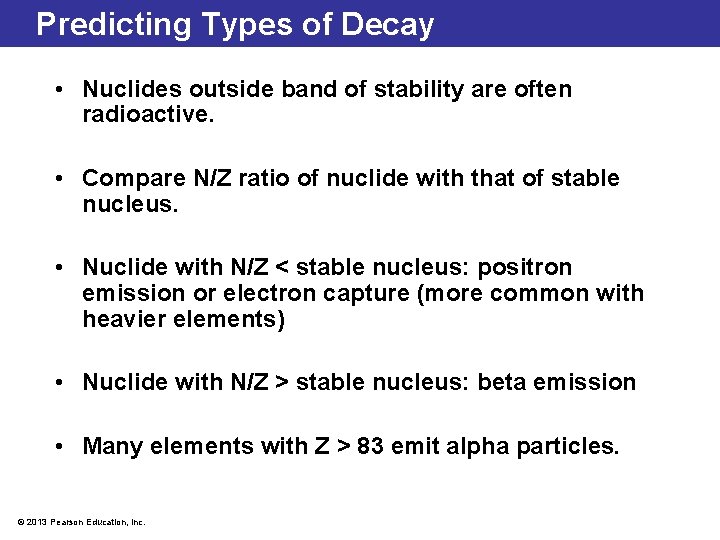
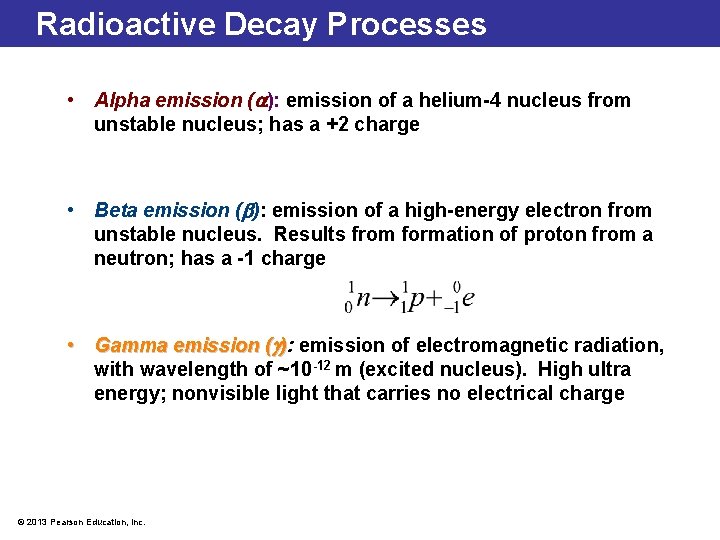
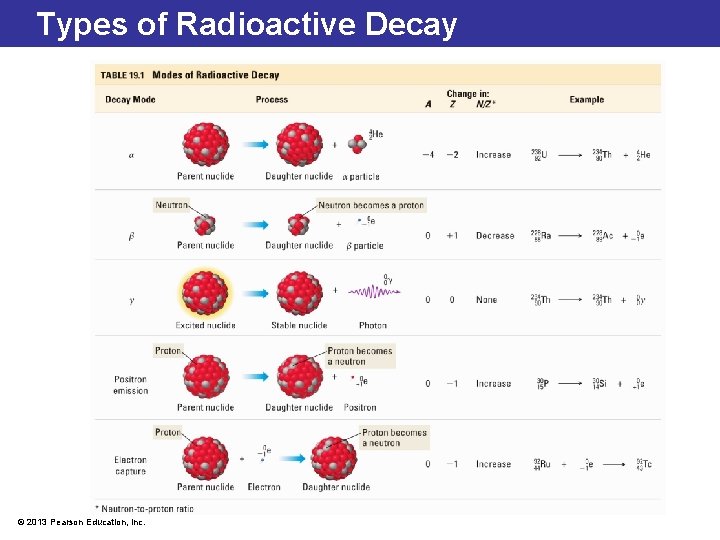
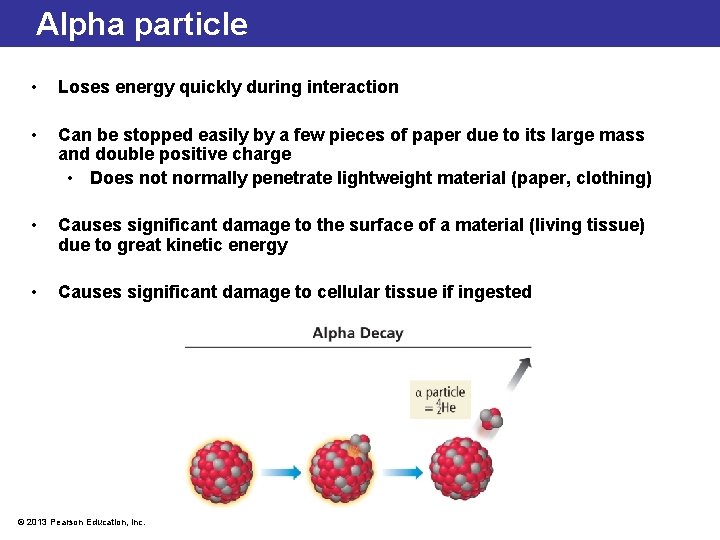
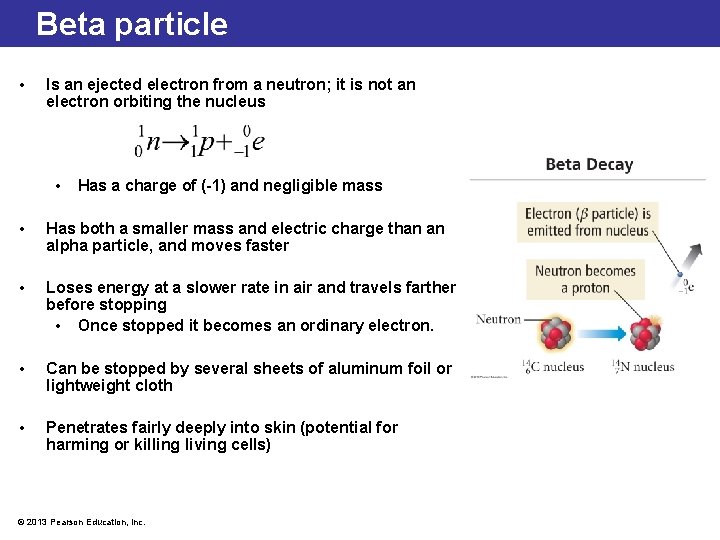
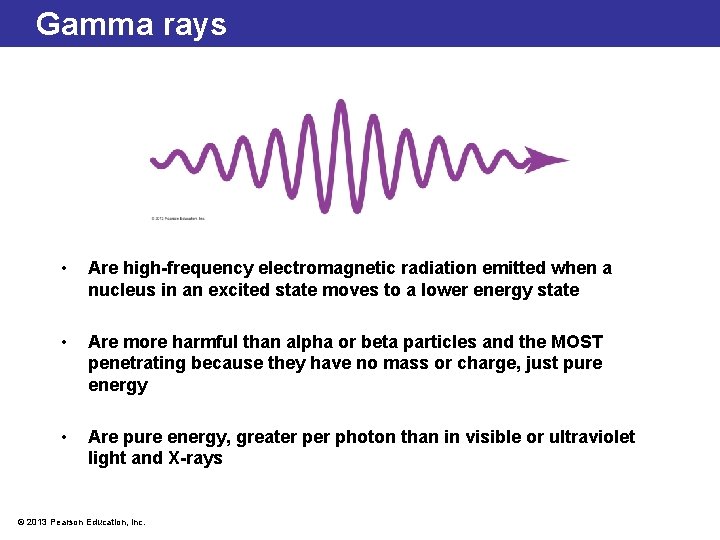
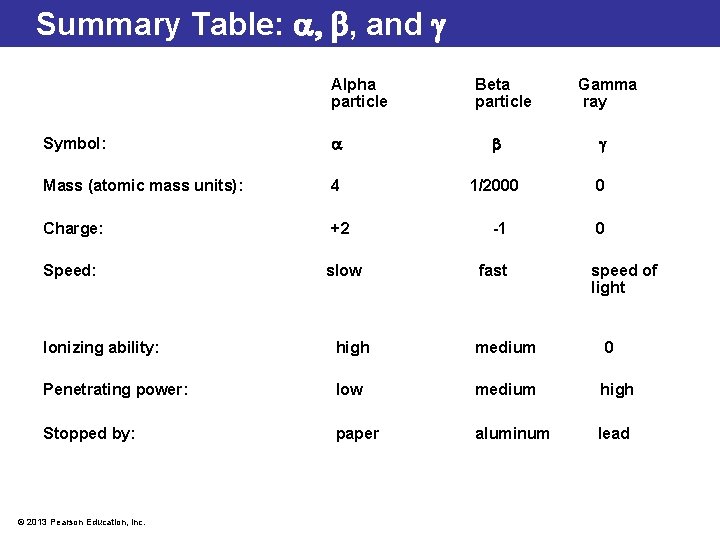
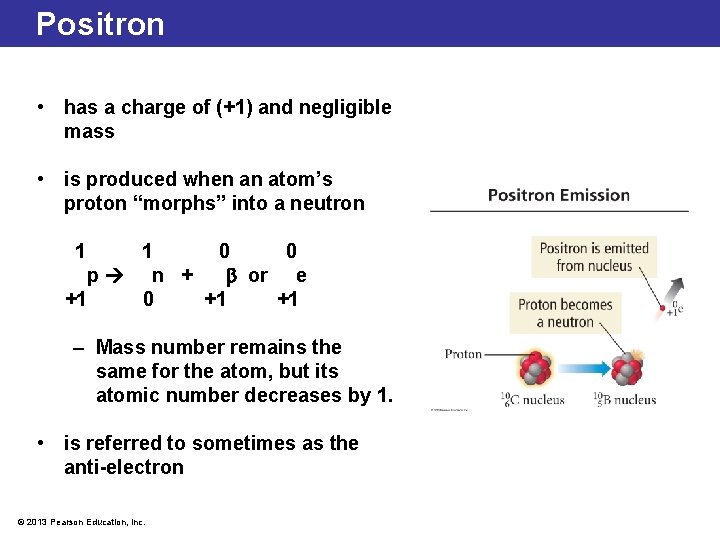
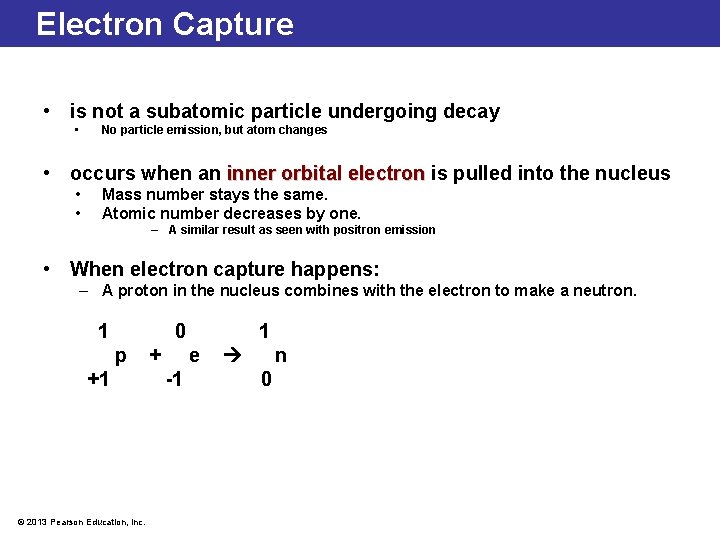
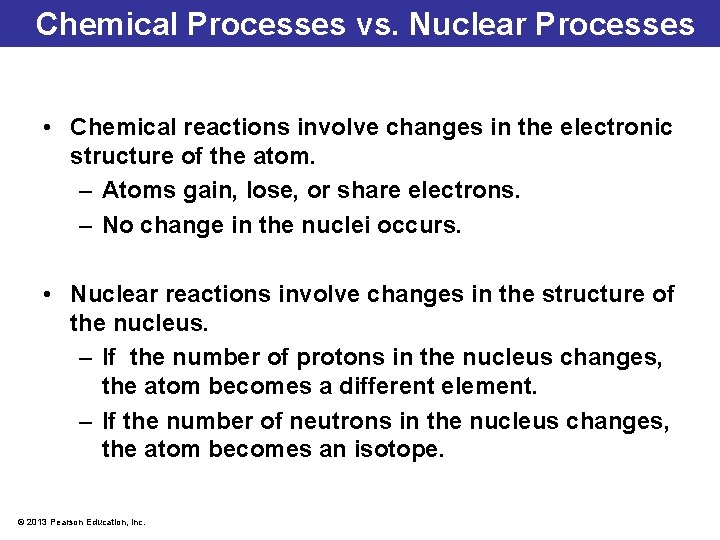
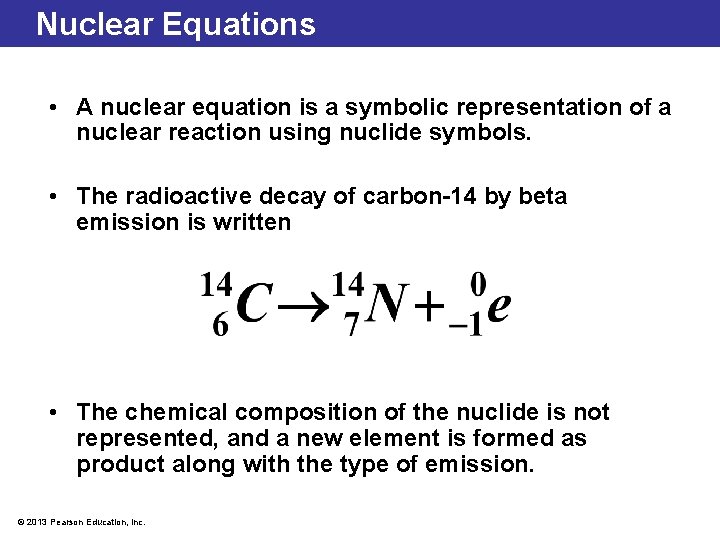
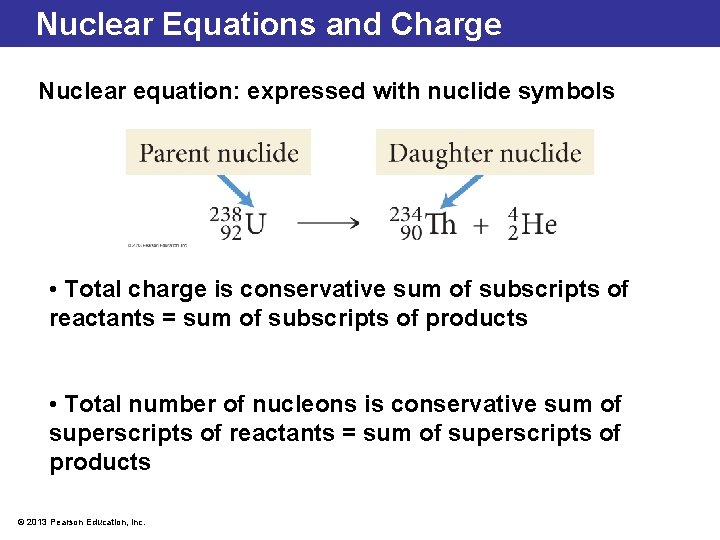
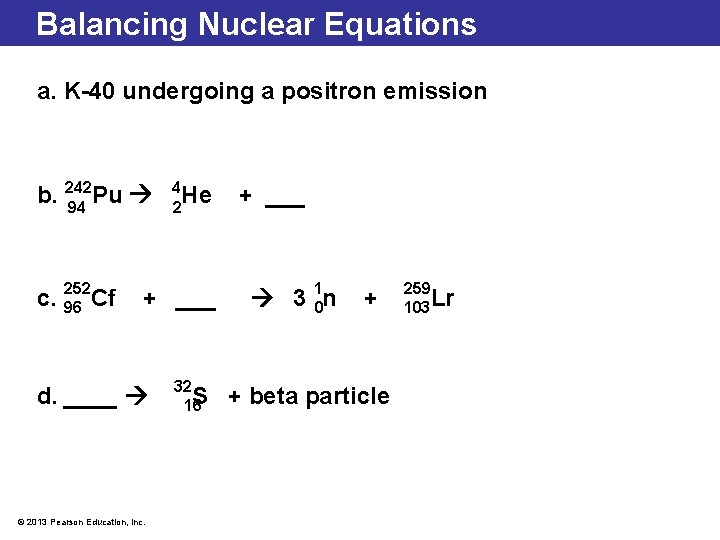
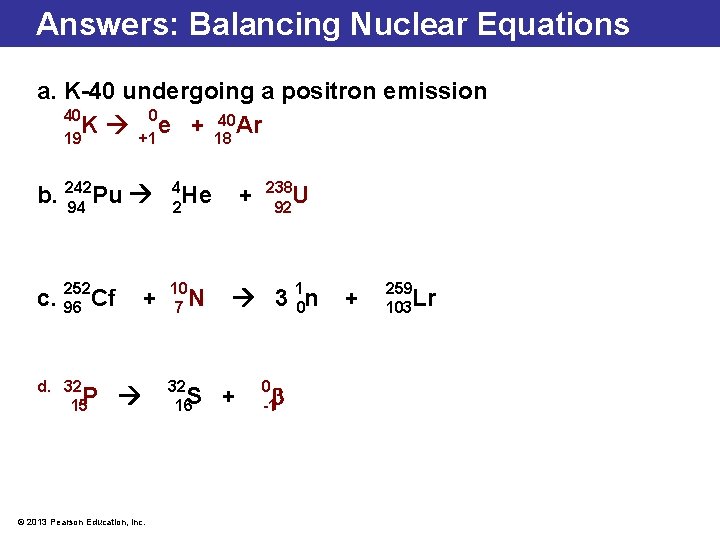
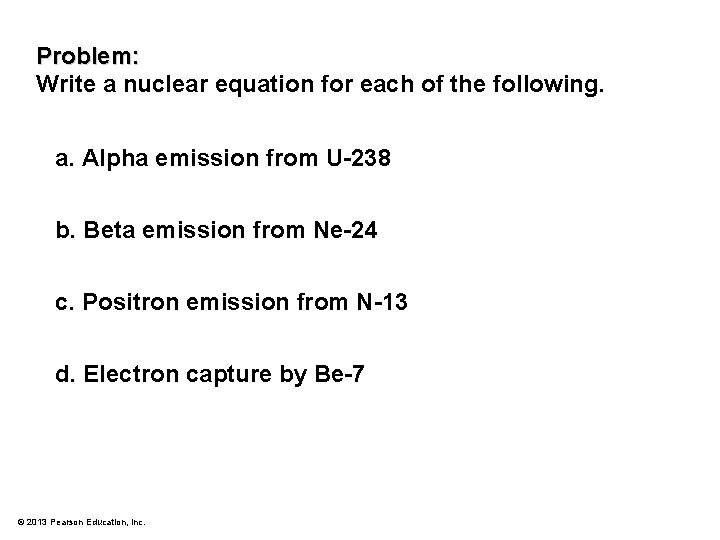
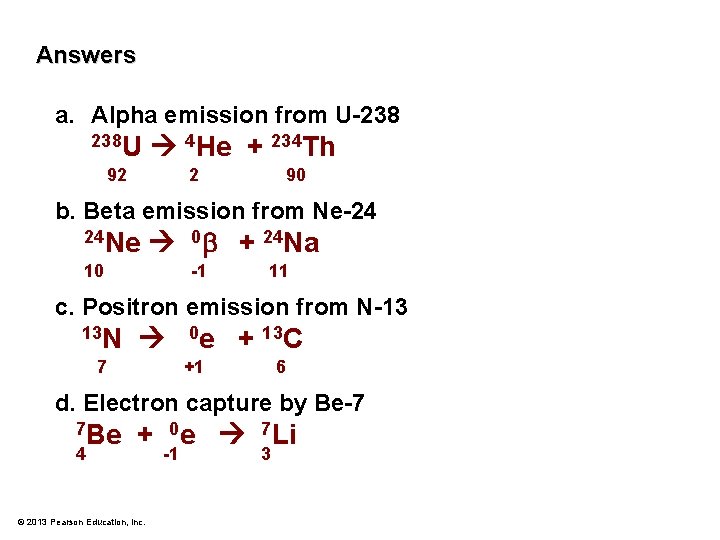
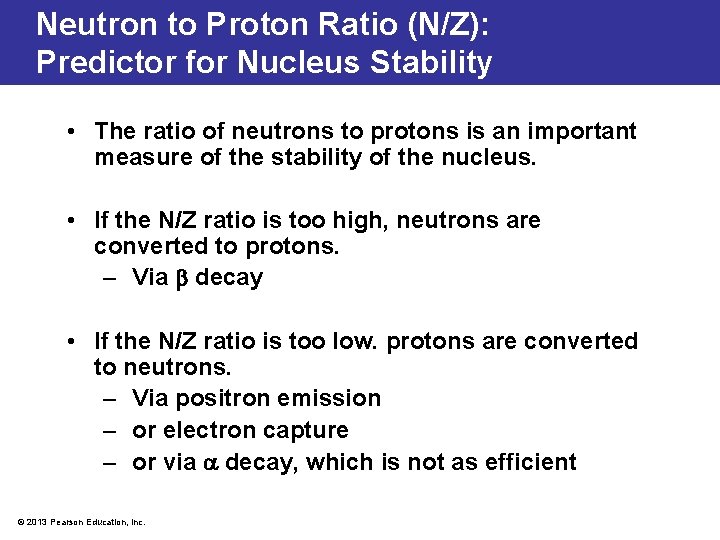
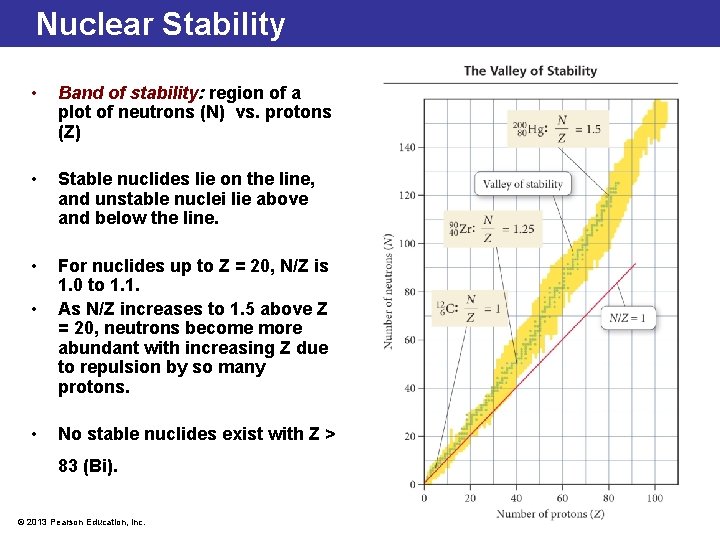
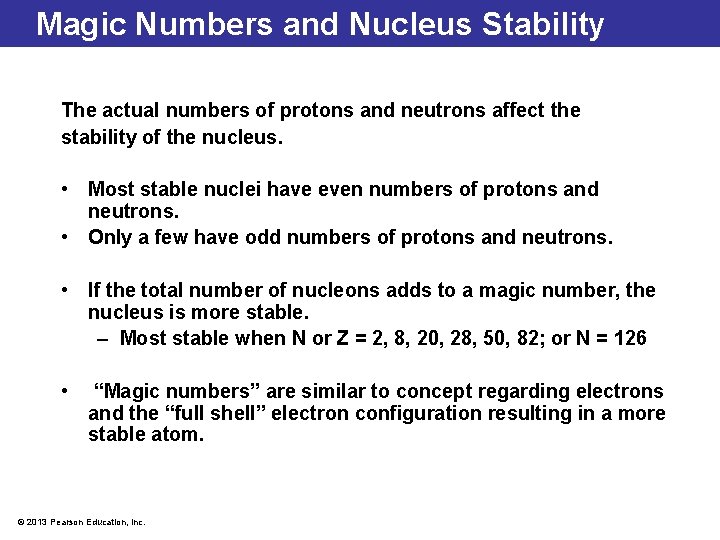
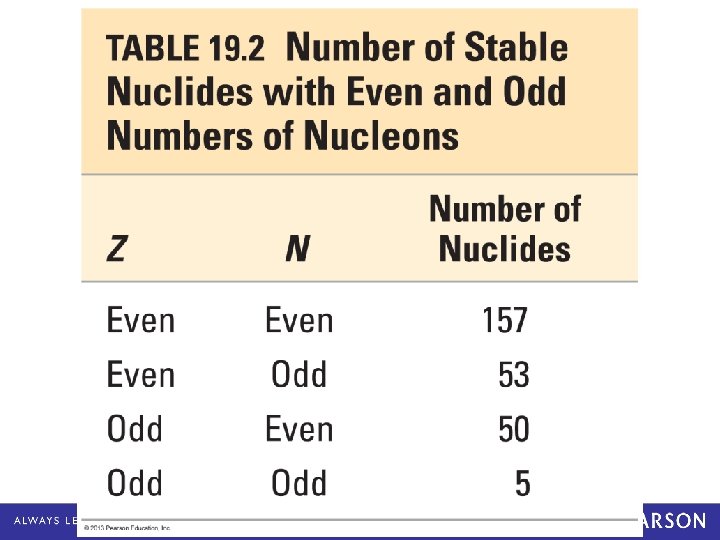
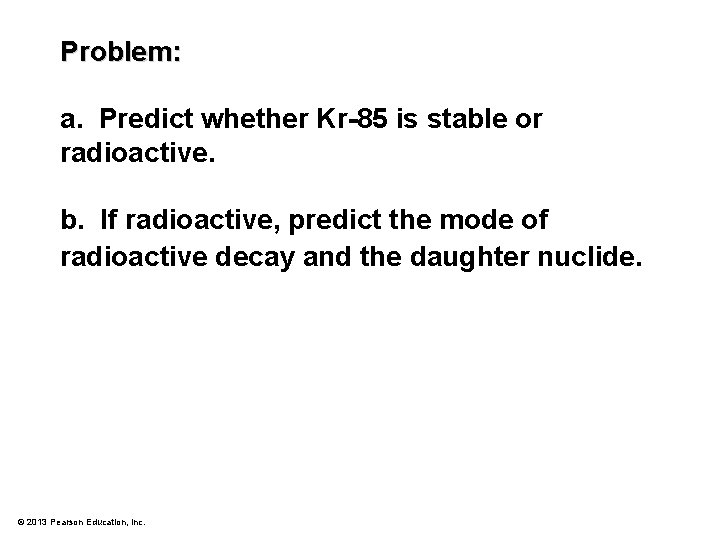
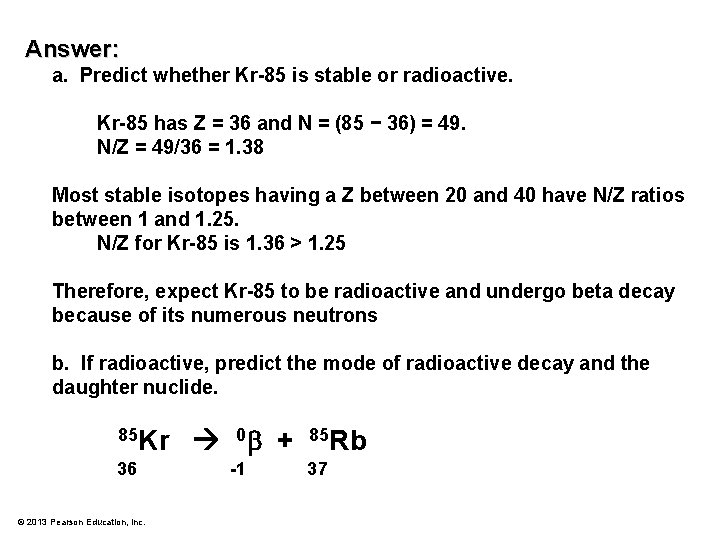
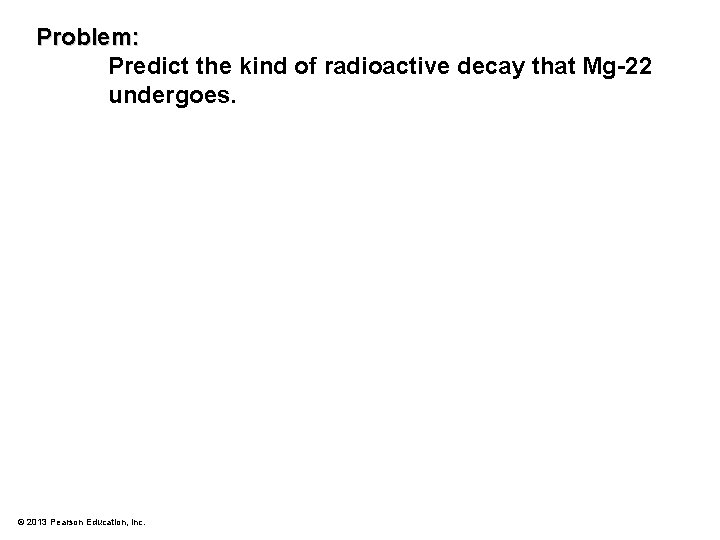
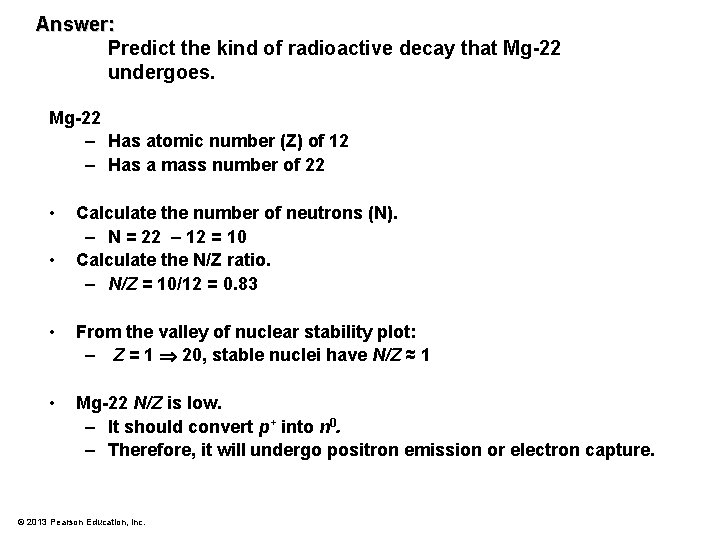
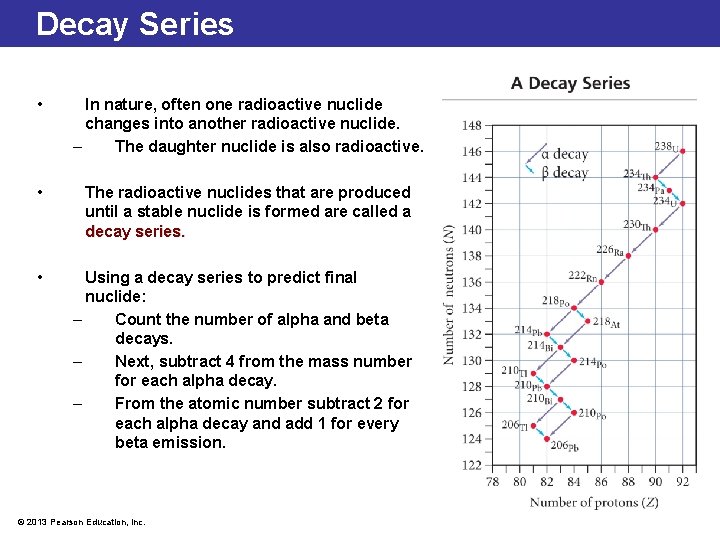
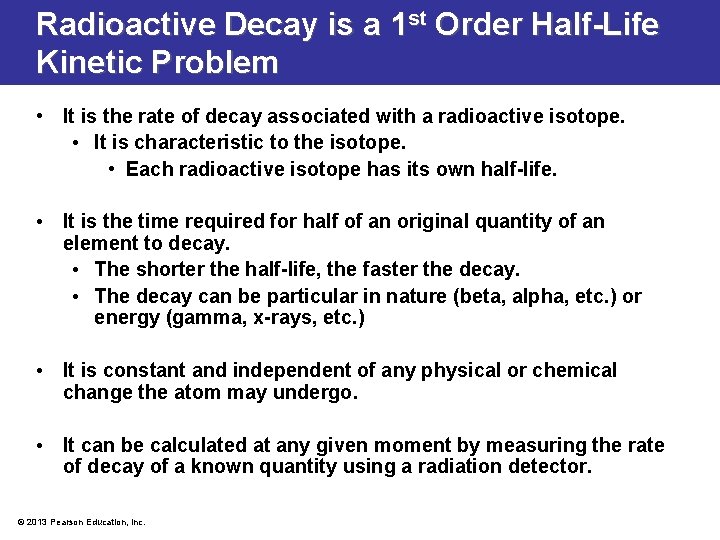
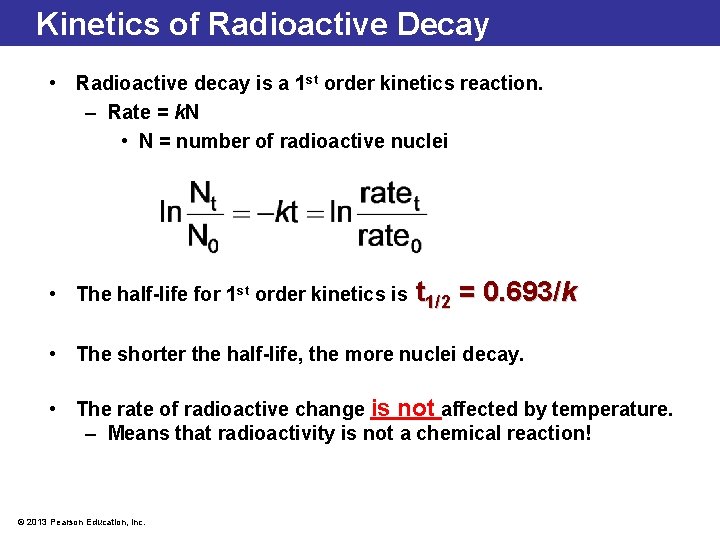
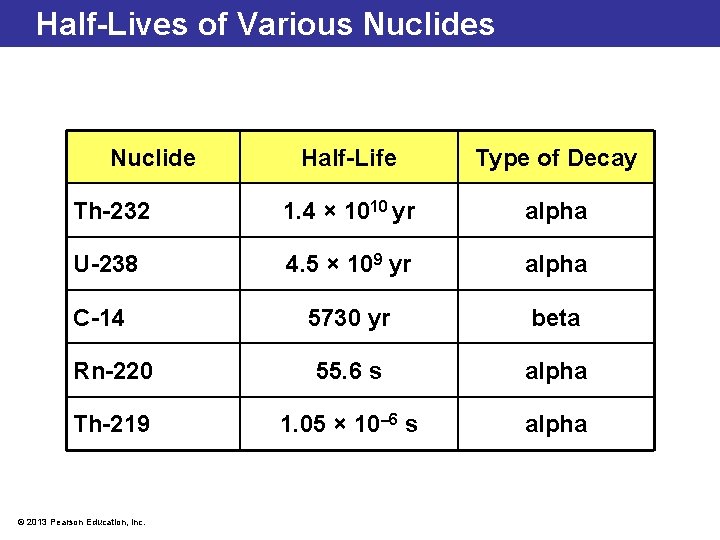
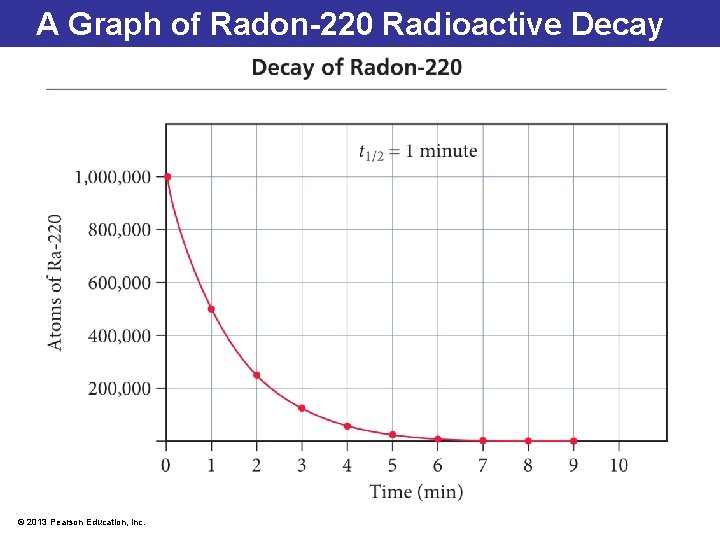
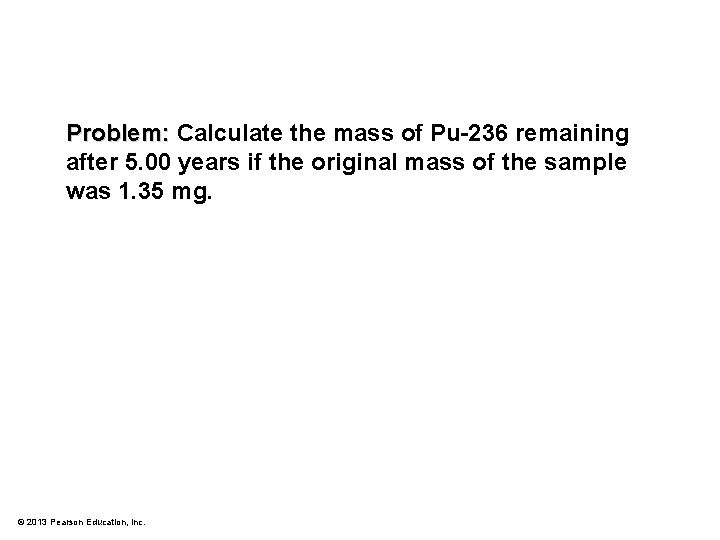
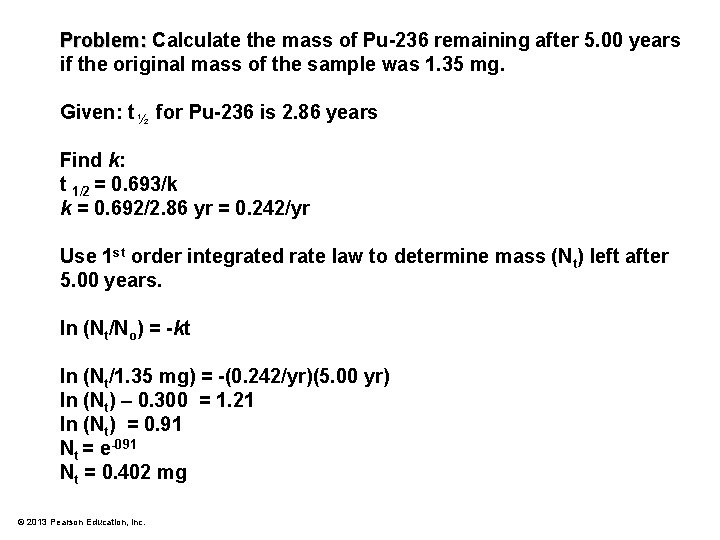
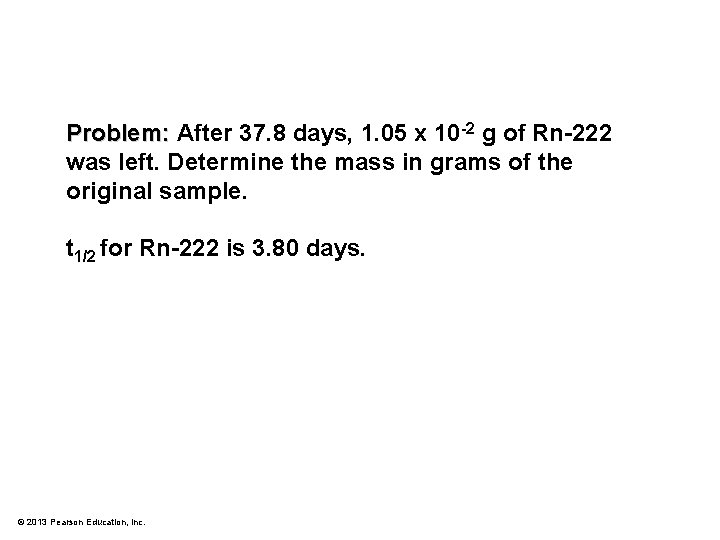
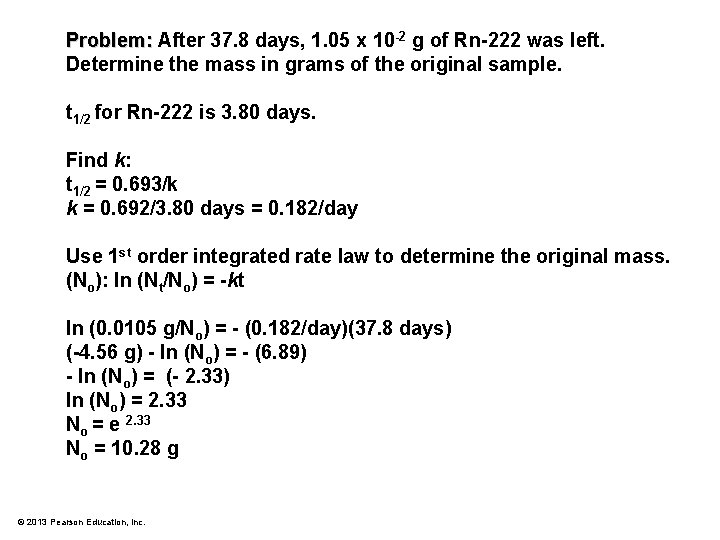
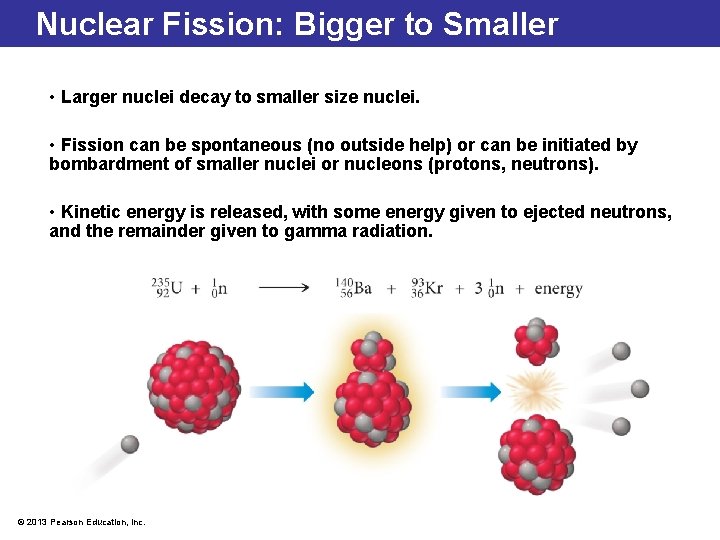
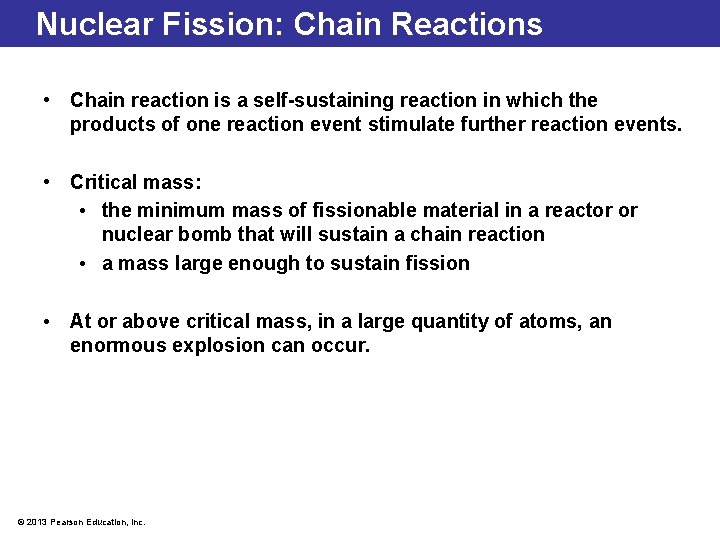
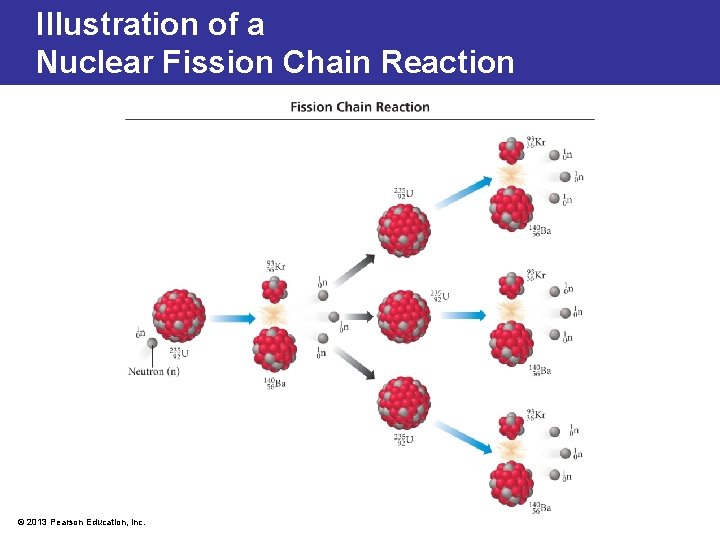
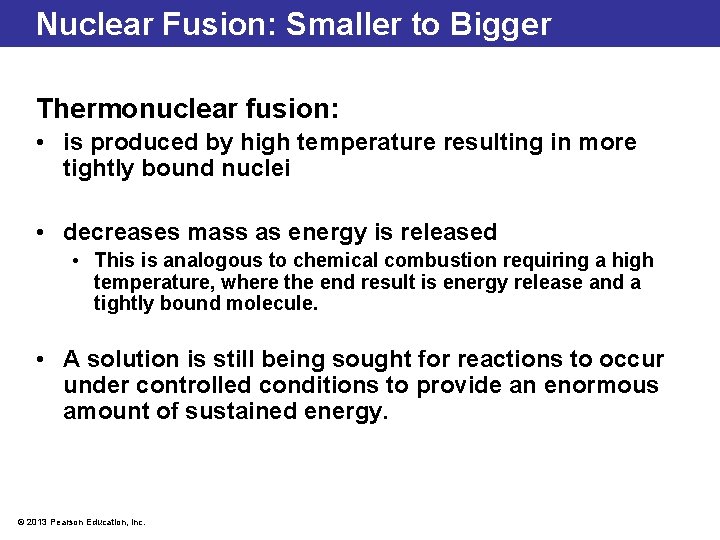
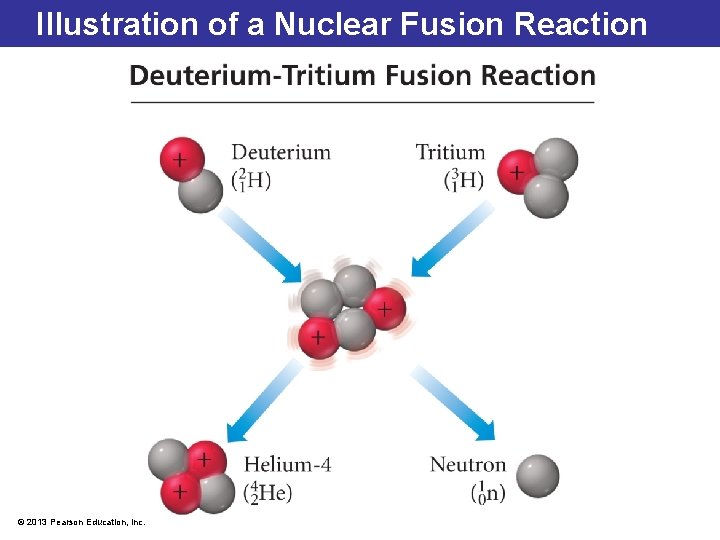
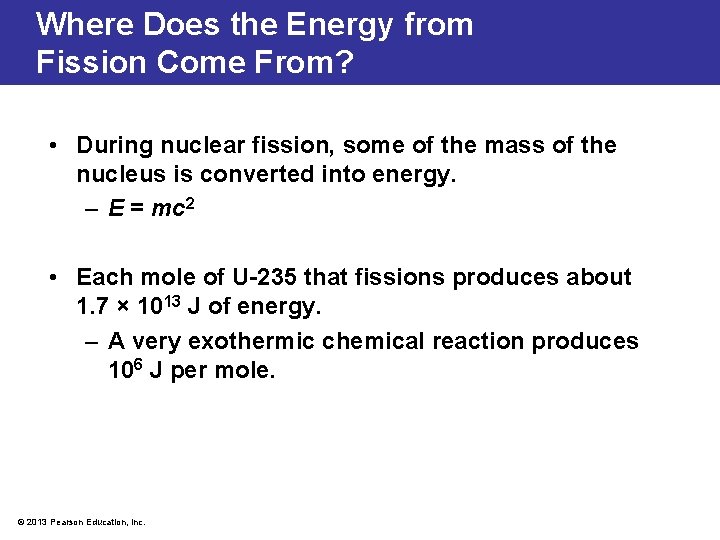
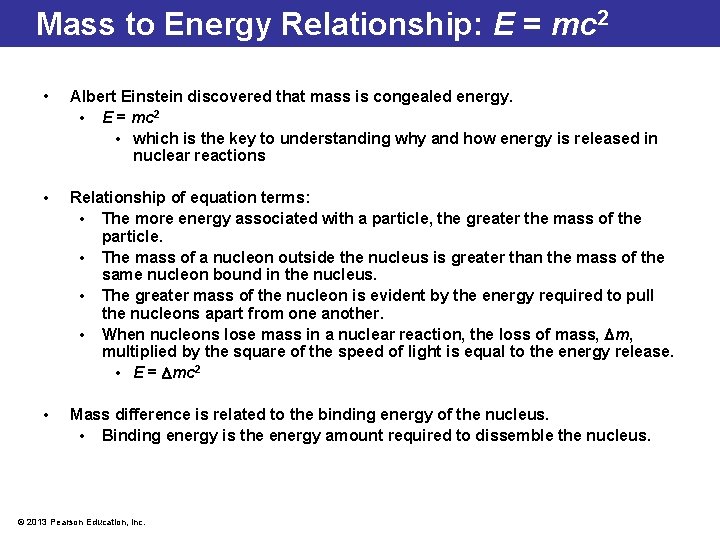
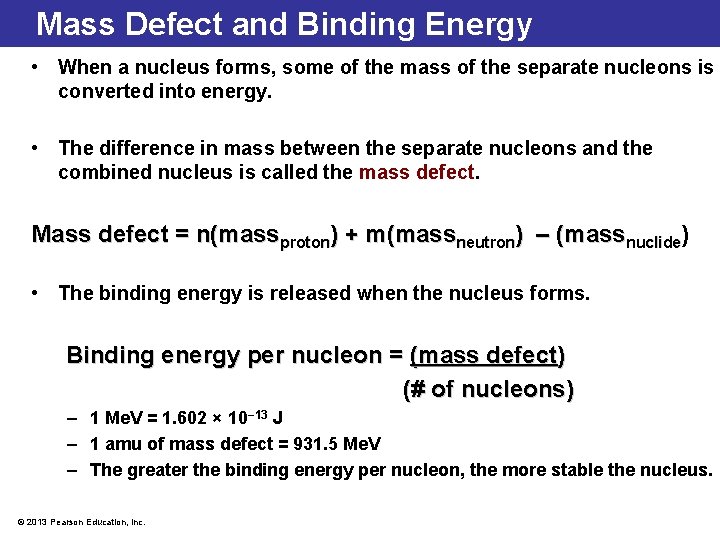
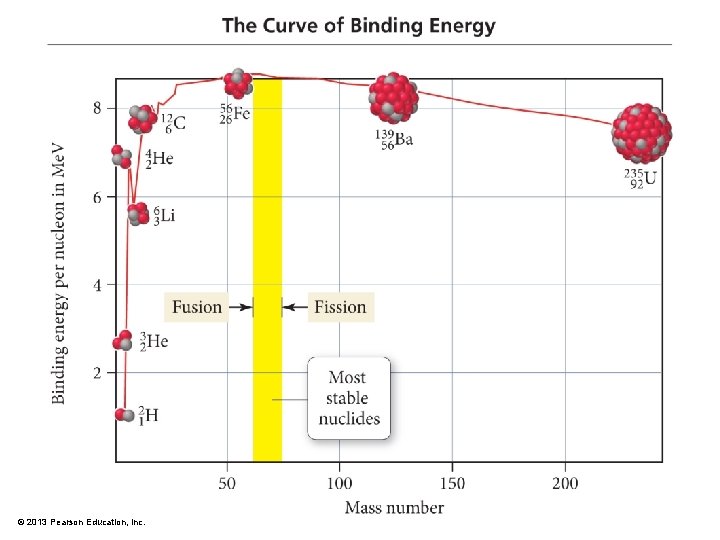
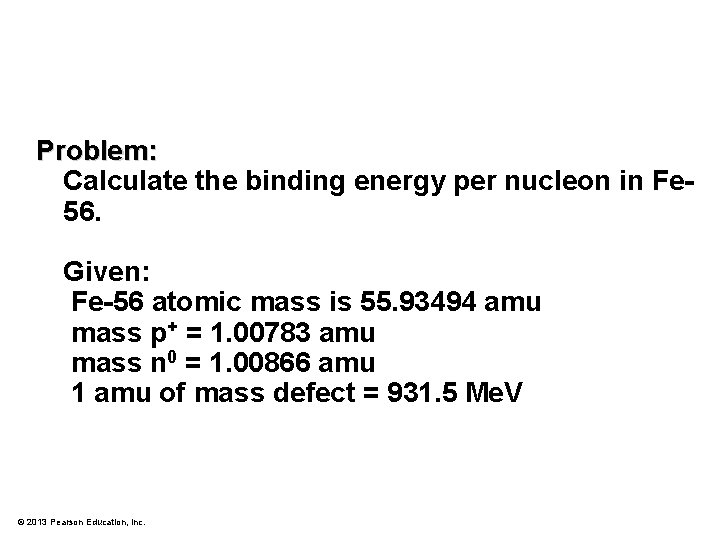
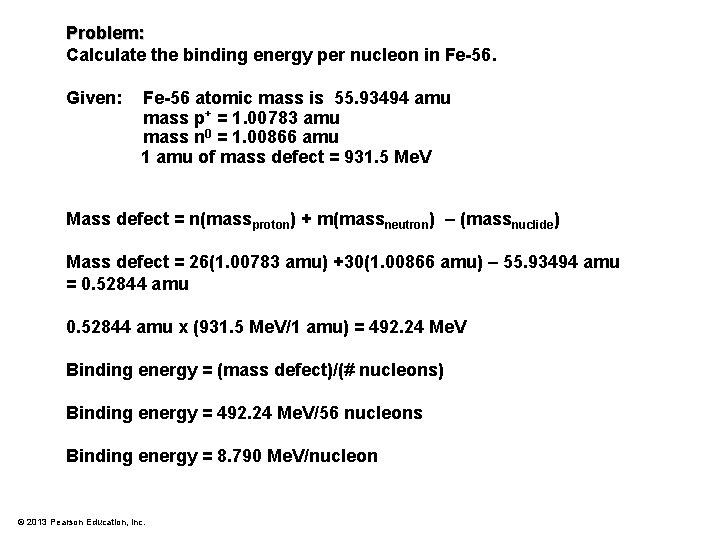
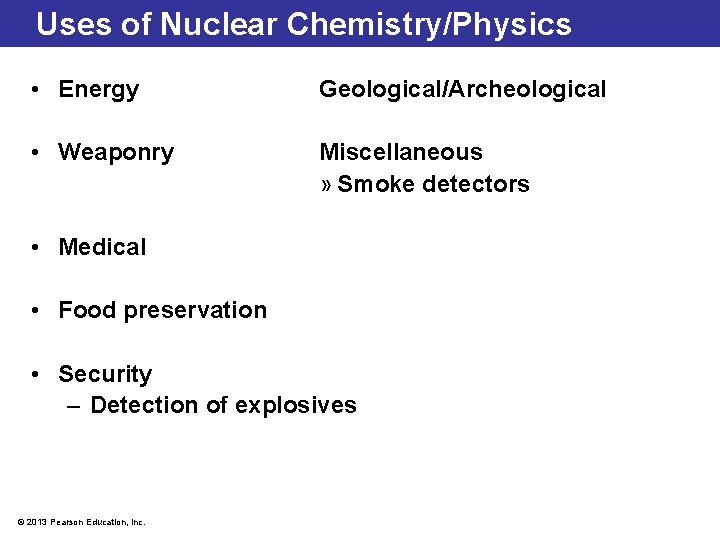
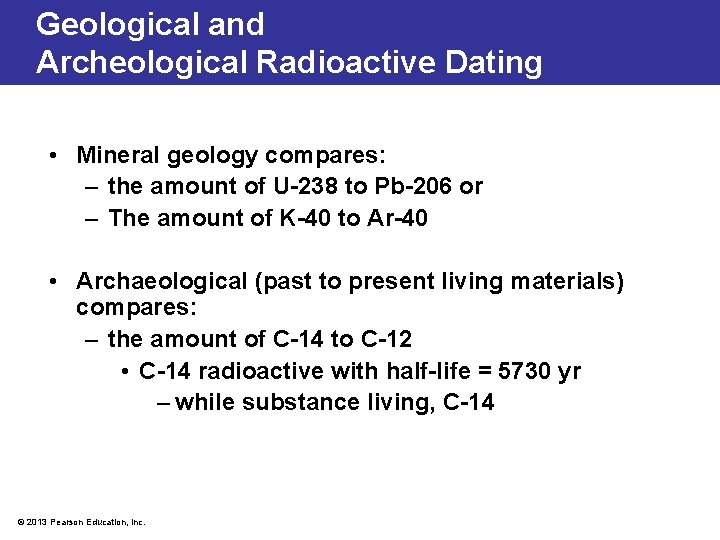
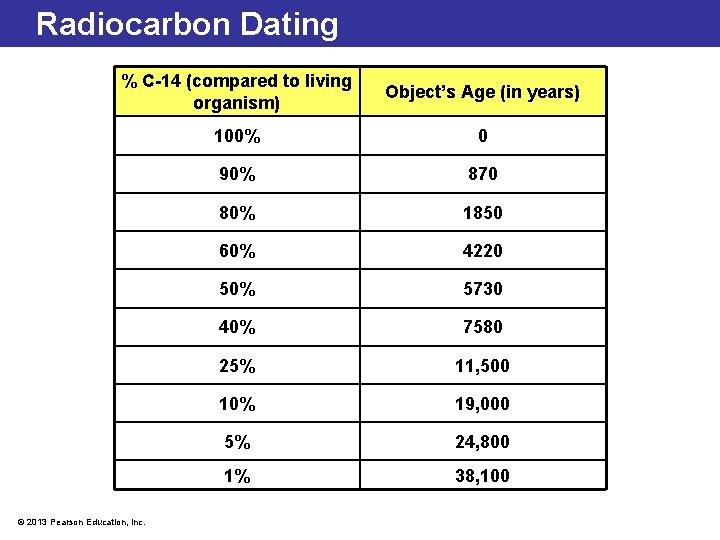
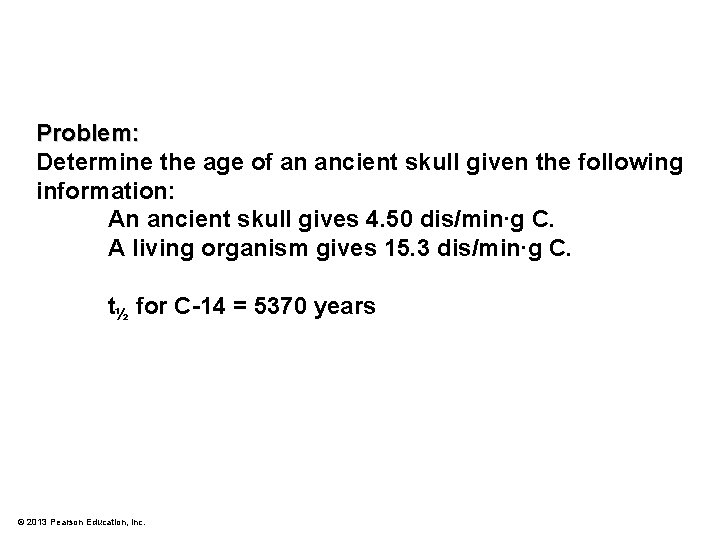
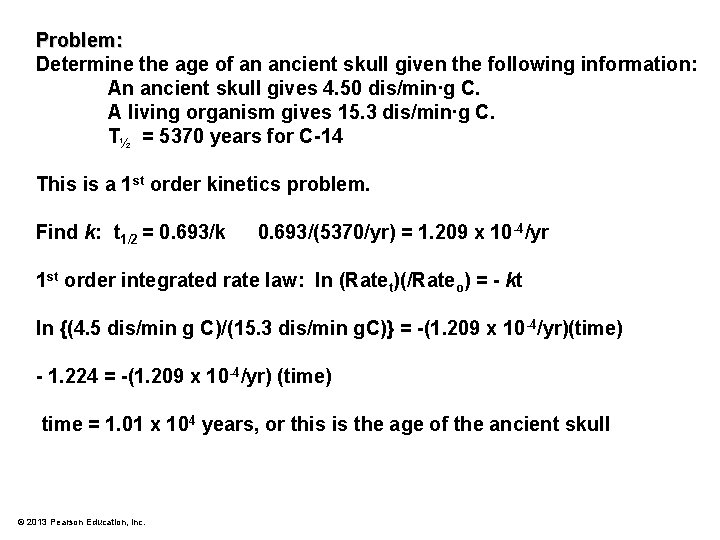
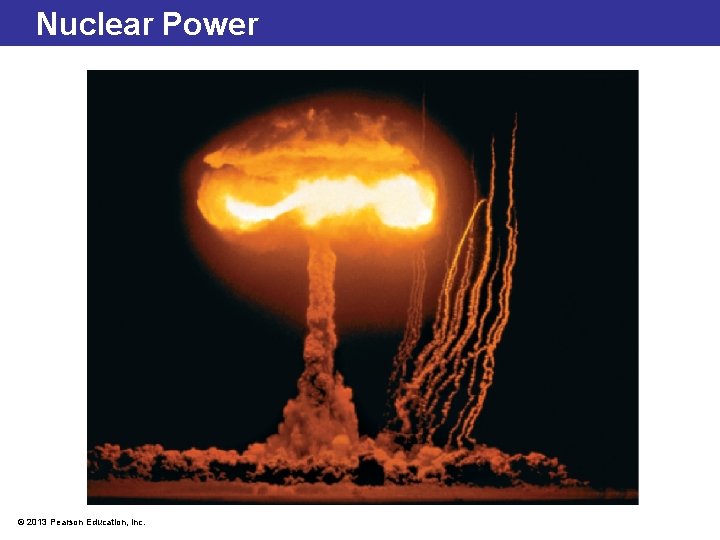
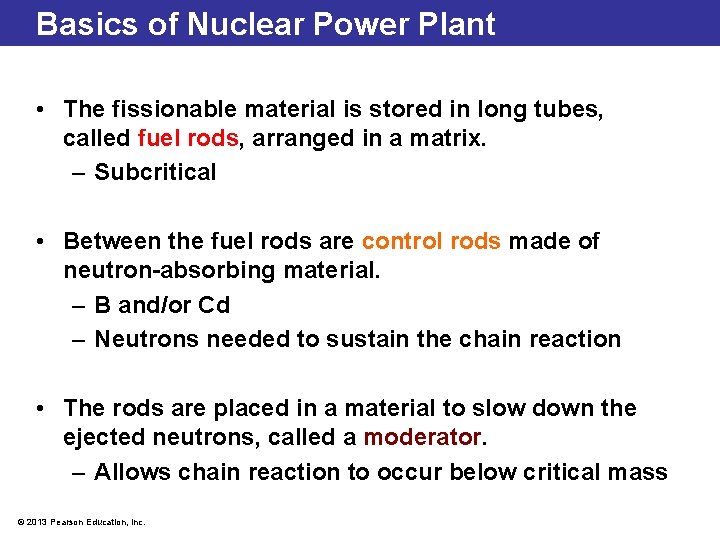
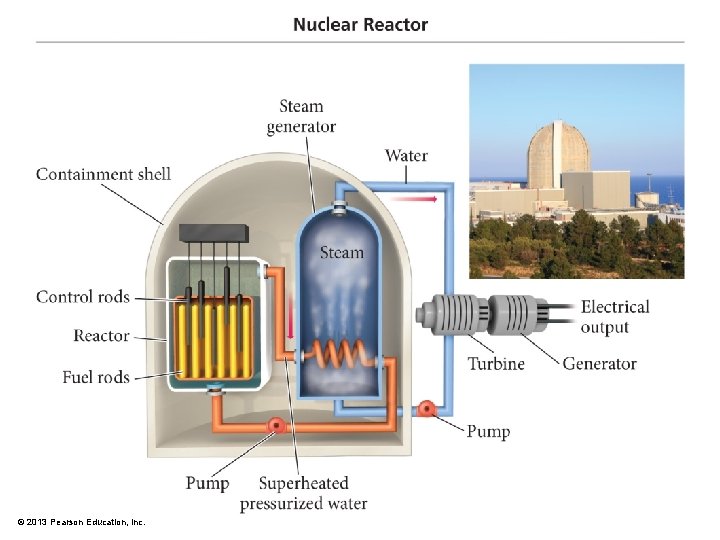
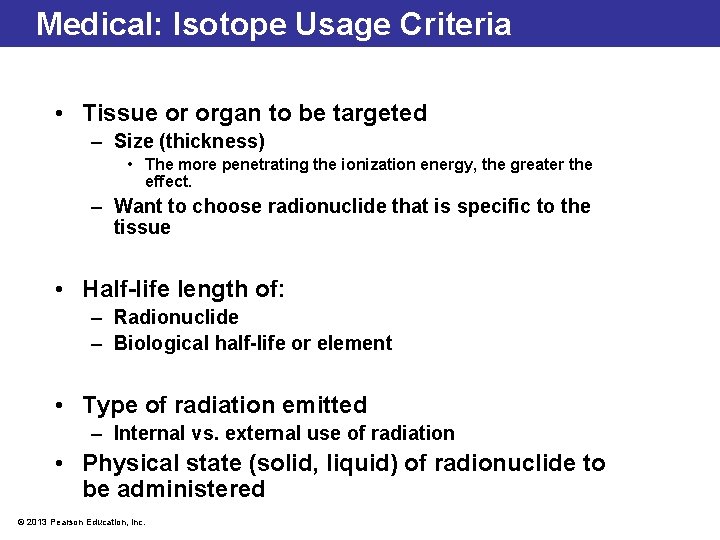
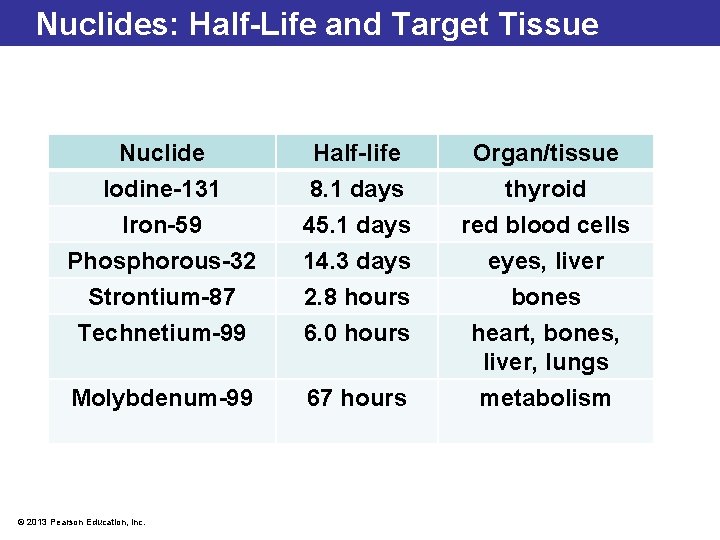
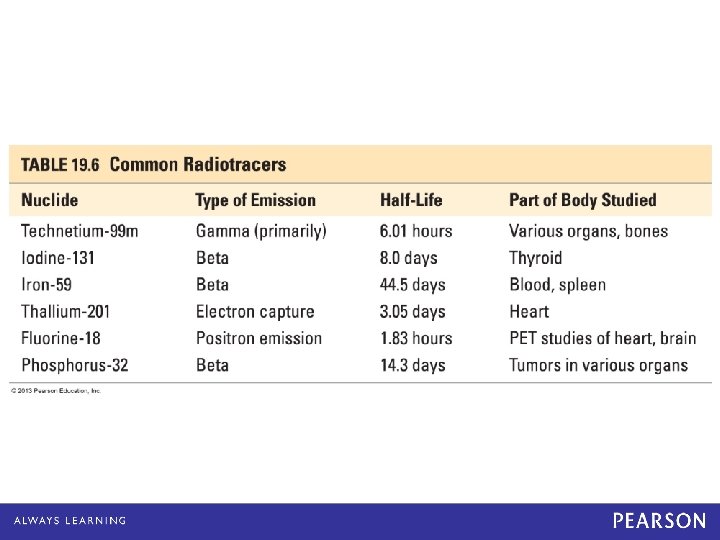
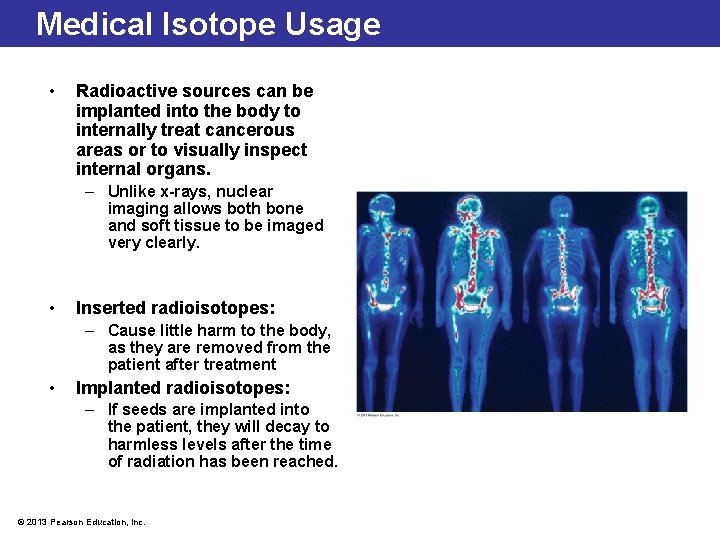
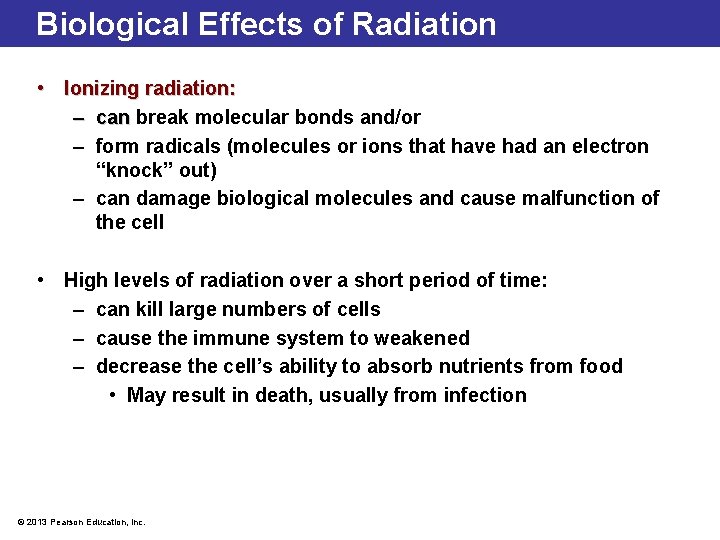
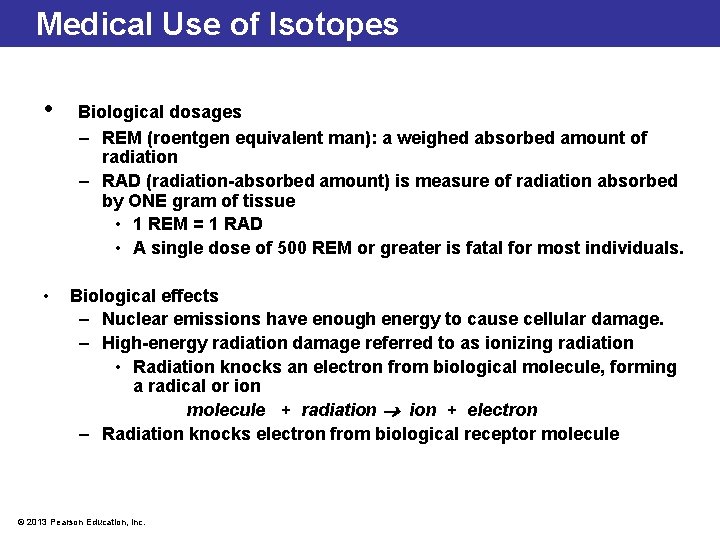
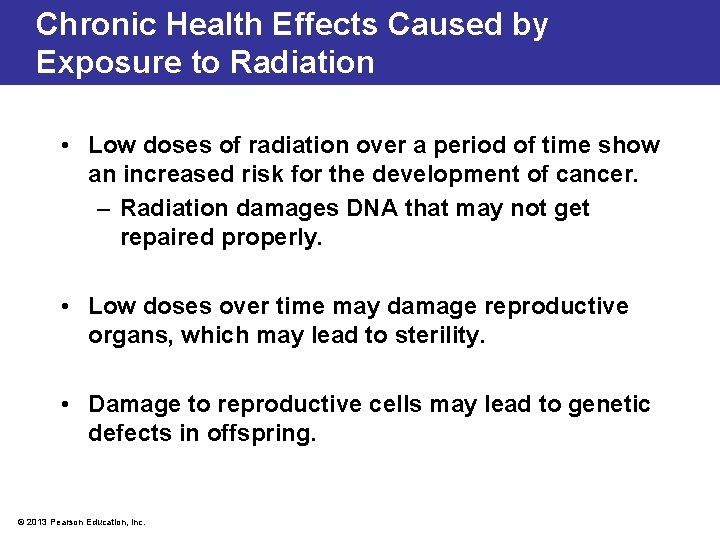
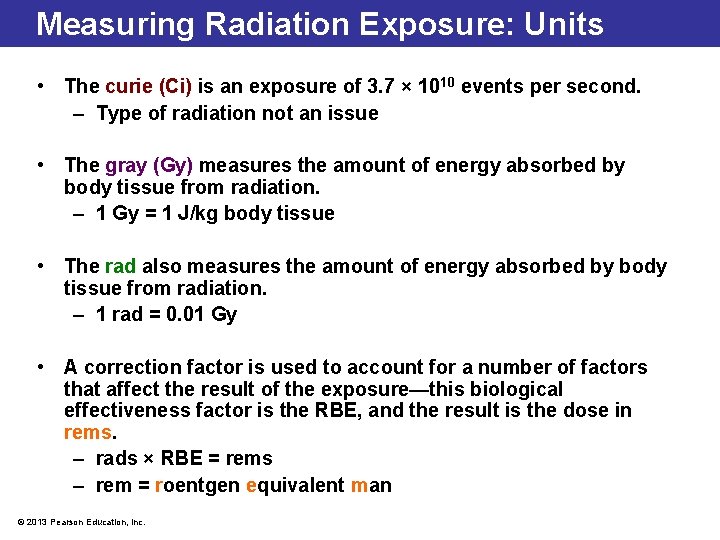
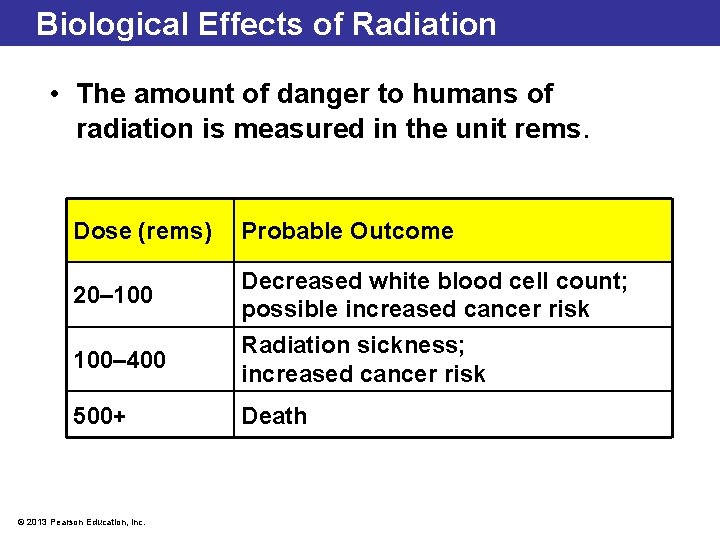
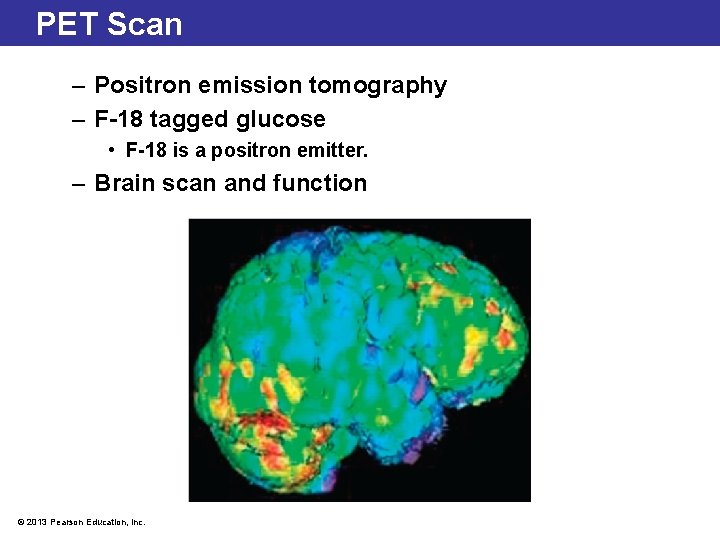
- Slides: 69
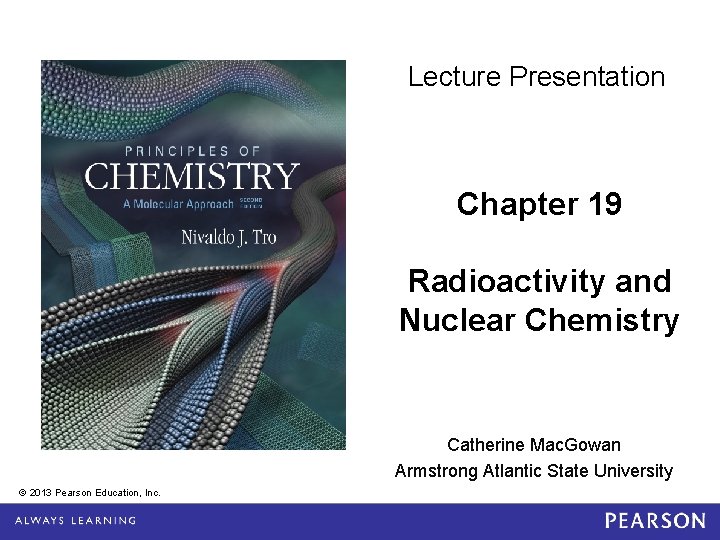
Lecture Presentation Chapter 19 Radioactivity and Nuclear Chemistry Catherine Mac. Gowan Armstrong Atlantic State University © 2013 Pearson Education, Inc.
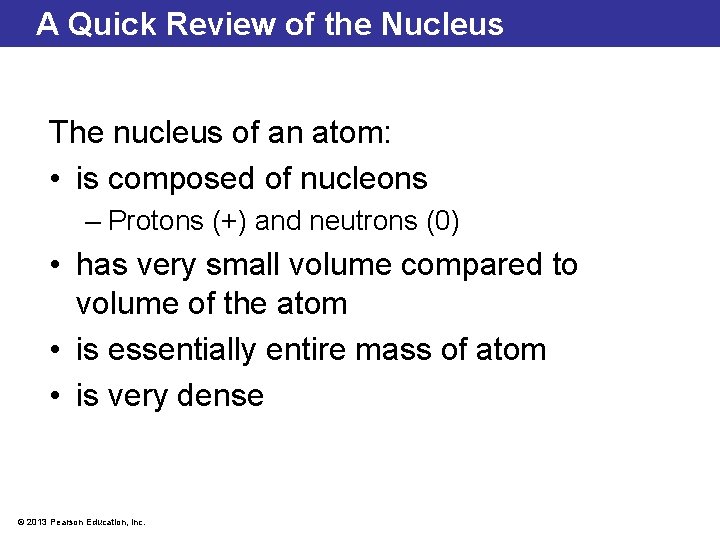
A Quick Review of the Nucleus The nucleus of an atom: • is composed of nucleons – Protons (+) and neutrons (0) • has very small volume compared to volume of the atom • is essentially entire mass of atom • is very dense © 2013 Pearson Education, Inc.
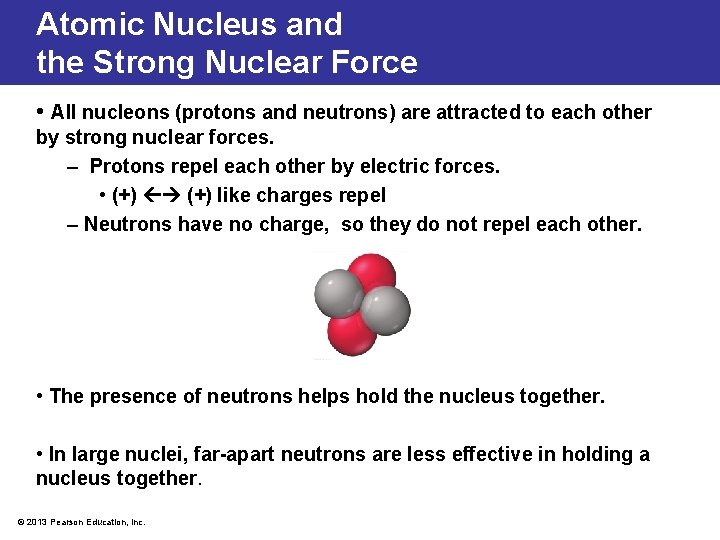
Atomic Nucleus and the Strong Nuclear Force • All nucleons (protons and neutrons) are attracted to each other by strong nuclear forces. – Protons repel each other by electric forces. • (+) like charges repel – Neutrons have no charge, so they do not repel each other. • The presence of neutrons helps hold the nucleus together. • In large nuclei, far-apart neutrons are less effective in holding a nucleus together. © 2013 Pearson Education, Inc.
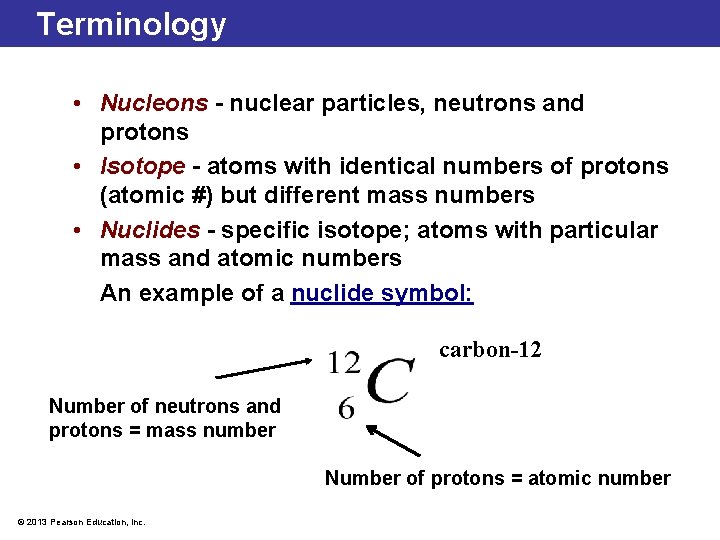
Terminology • Nucleons - nuclear particles, neutrons and protons • Isotope - atoms with identical numbers of protons (atomic #) but different mass numbers • Nuclides - specific isotope; atoms with particular mass and atomic numbers An example of a nuclide symbol: carbon-12 Number of neutrons and protons = mass number Number of protons = atomic number © 2013 Pearson Education, Inc.
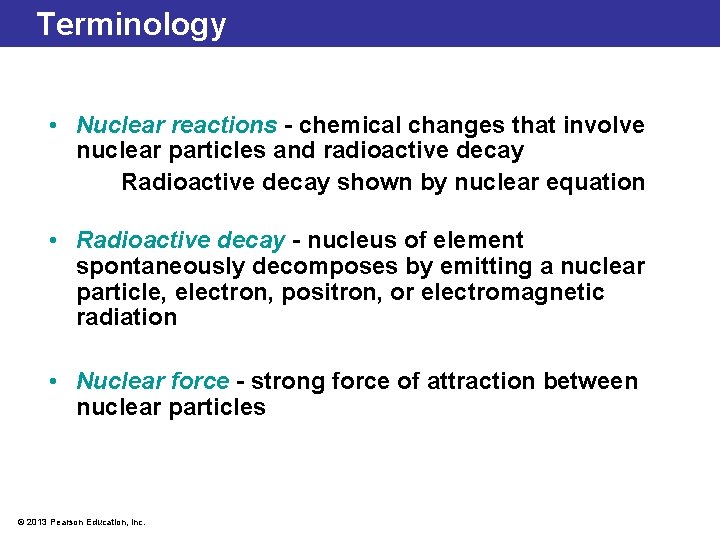
Terminology • Nuclear reactions - chemical changes that involve nuclear particles and radioactive decay Radioactive decay shown by nuclear equation • Radioactive decay - nucleus of element spontaneously decomposes by emitting a nuclear particle, electron, positron, or electromagnetic radiation • Nuclear force - strong force of attraction between nuclear particles © 2013 Pearson Education, Inc.
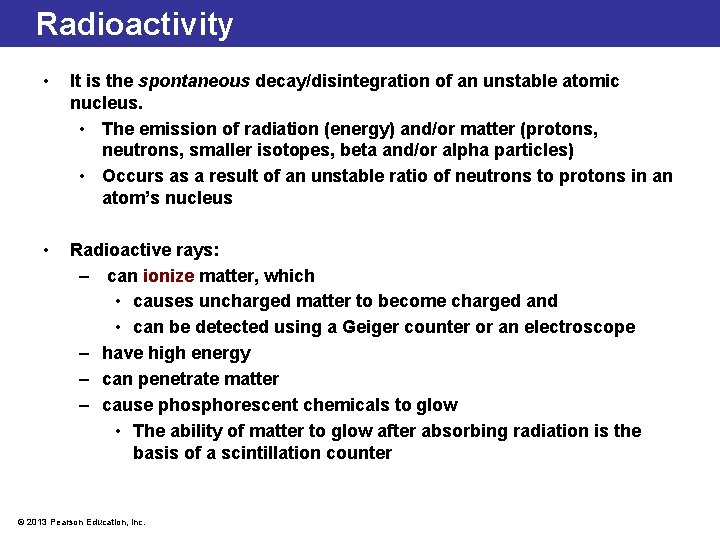
Radioactivity • It is the spontaneous decay/disintegration of an unstable atomic nucleus. • The emission of radiation (energy) and/or matter (protons, neutrons, smaller isotopes, beta and/or alpha particles) • Occurs as a result of an unstable ratio of neutrons to protons in an atom’s nucleus • Radioactive rays: – can ionize matter, which • causes uncharged matter to become charged and • can be detected using a Geiger counter or an electroscope – have high energy – can penetrate matter – cause phosphorescent chemicals to glow • The ability of matter to glow after absorbing radiation is the basis of a scintillation counter © 2013 Pearson Education, Inc.
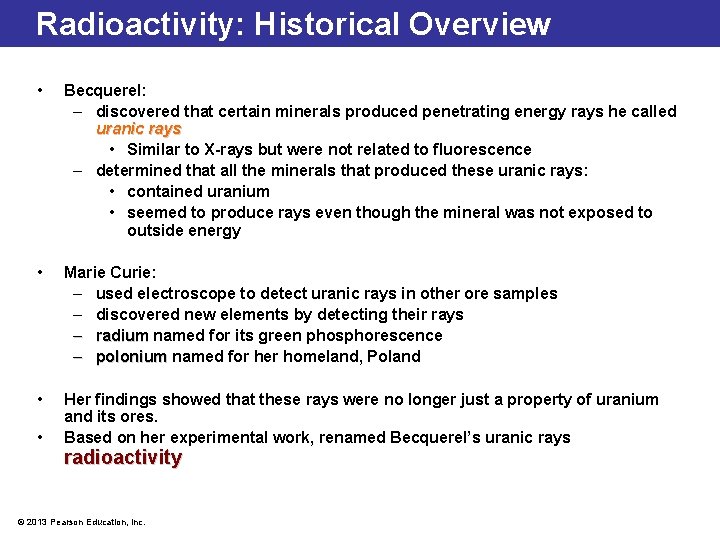
Radioactivity: Historical Overview • Becquerel: – discovered that certain minerals produced penetrating energy rays he called uranic rays • Similar to X-rays but were not related to fluorescence – determined that all the minerals that produced these uranic rays: • contained uranium • seemed to produce rays even though the mineral was not exposed to outside energy • Marie Curie: – used electroscope to detect uranic rays in other ore samples – discovered new elements by detecting their rays – radium named for its green phosphorescence – polonium named for her homeland, Poland • Her findings showed that these rays were no longer just a property of uranium and its ores. Based on her experimental work, renamed Becquerel’s uranic rays • radioactivity © 2013 Pearson Education, Inc.
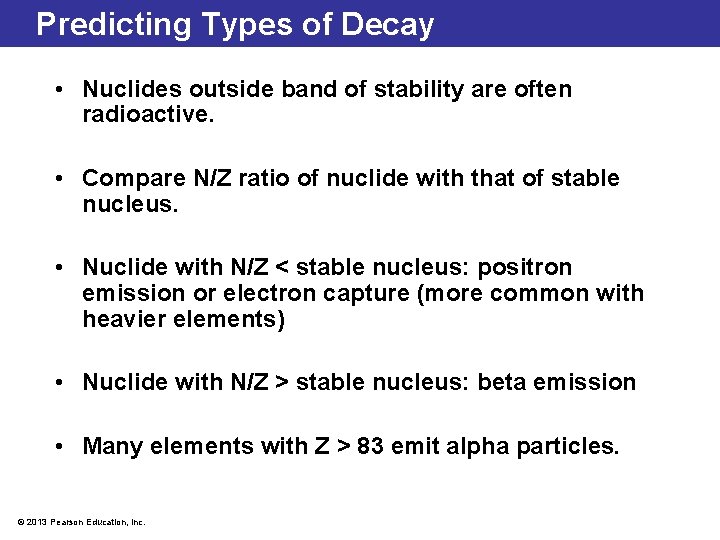
Predicting Types of Decay • Nuclides outside band of stability are often radioactive. • Compare N/Z ratio of nuclide with that of stable nucleus. • Nuclide with N/Z < stable nucleus: positron emission or electron capture (more common with heavier elements) • Nuclide with N/Z > stable nucleus: beta emission • Many elements with Z > 83 emit alpha particles. © 2013 Pearson Education, Inc.
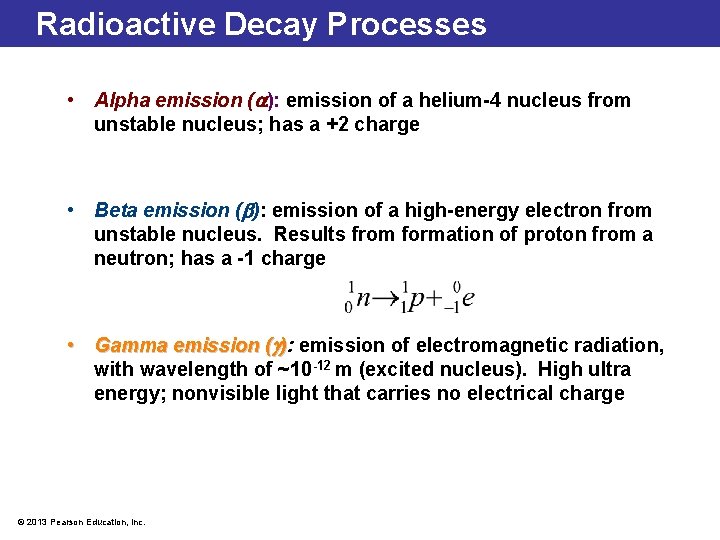
Radioactive Decay Processes • Alpha emission ( ): emission of a helium-4 nucleus from unstable nucleus; has a +2 charge • Beta emission ( ): emission of a high-energy electron from unstable nucleus. Results from formation of proton from a neutron; has a -1 charge • Gamma emission ( ): emission of electromagnetic radiation, with wavelength of ~10 -12 m (excited nucleus). High ultra energy; nonvisible light that carries no electrical charge © 2013 Pearson Education, Inc.
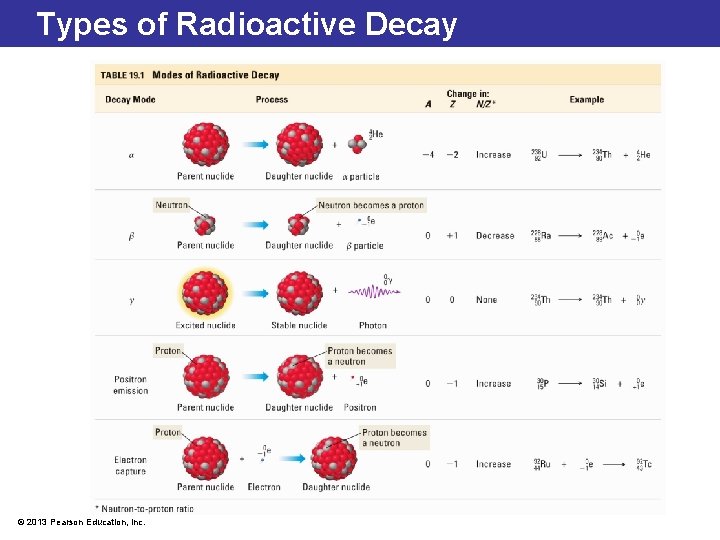
Types of Radioactive Decay © 2013 Pearson Education, Inc.
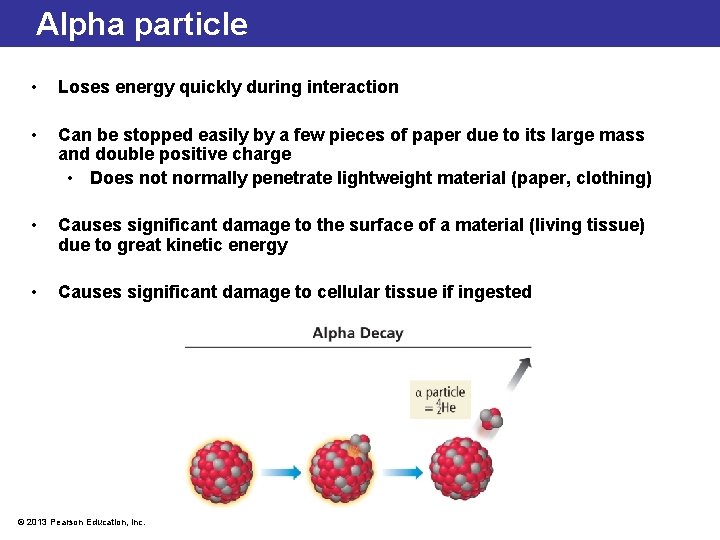
Alpha particle • Loses energy quickly during interaction • Can be stopped easily by a few pieces of paper due to its large mass and double positive charge • Does not normally penetrate lightweight material (paper, clothing) • Causes significant damage to the surface of a material (living tissue) due to great kinetic energy • Causes significant damage to cellular tissue if ingested © 2013 Pearson Education, Inc.
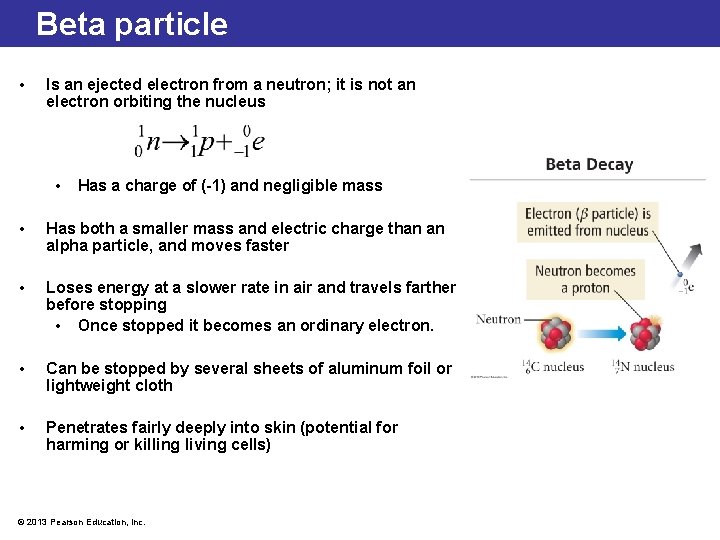
Beta particle • Is an ejected electron from a neutron; it is not an electron orbiting the nucleus • Has a charge of (-1) and negligible mass • Has both a smaller mass and electric charge than an alpha particle, and moves faster • Loses energy at a slower rate in air and travels farther before stopping • Once stopped it becomes an ordinary electron. • Can be stopped by several sheets of aluminum foil or lightweight cloth • Penetrates fairly deeply into skin (potential for harming or killing living cells) © 2013 Pearson Education, Inc.
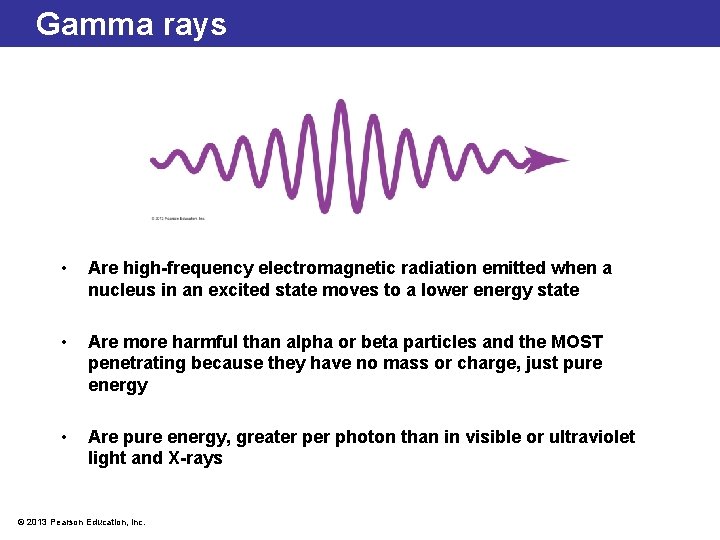
Gamma rays • Are high-frequency electromagnetic radiation emitted when a nucleus in an excited state moves to a lower energy state • Are more harmful than alpha or beta particles and the MOST penetrating because they have no mass or charge, just pure energy • Are pure energy, greater photon than in visible or ultraviolet light and X-rays © 2013 Pearson Education, Inc.
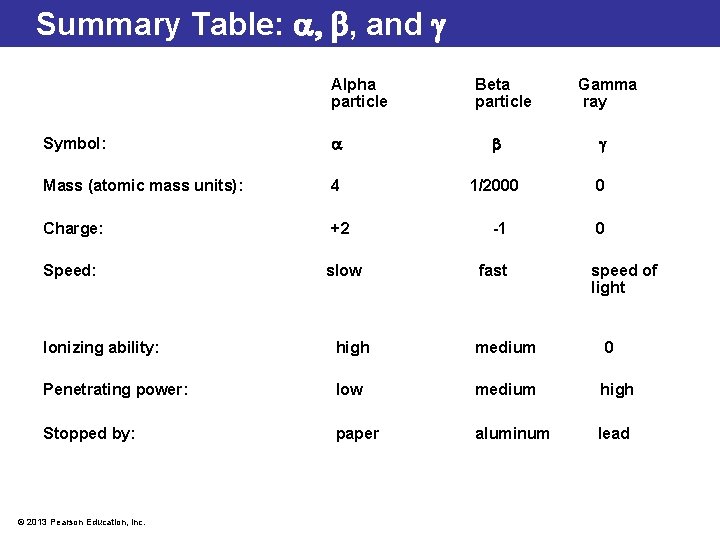
Summary Table: , and Alpha particle Beta particle Symbol: Mass (atomic mass units): 4 Charge: +2 Speed: slow Gamma ray 1/2000 -1 fast 0 0 speed of light Ionizing ability: high medium 0 Penetrating power: low medium high Stopped by: paper aluminum lead © 2013 Pearson Education, Inc.
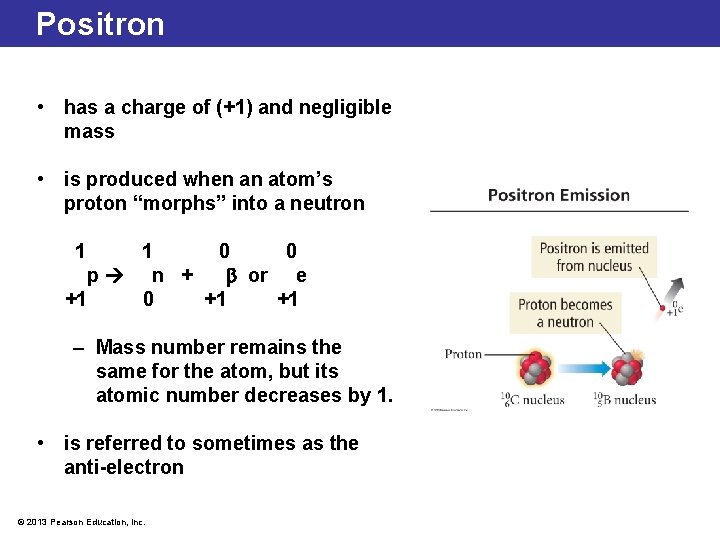
Positron • has a charge of (+1) and negligible mass • is produced when an atom’s proton “morphs” into a neutron 1 1 0 0 p n + or e +1 0 +1 +1 – Mass number remains the same for the atom, but its atomic number decreases by 1. • is referred to sometimes as the anti-electron © 2013 Pearson Education, Inc.
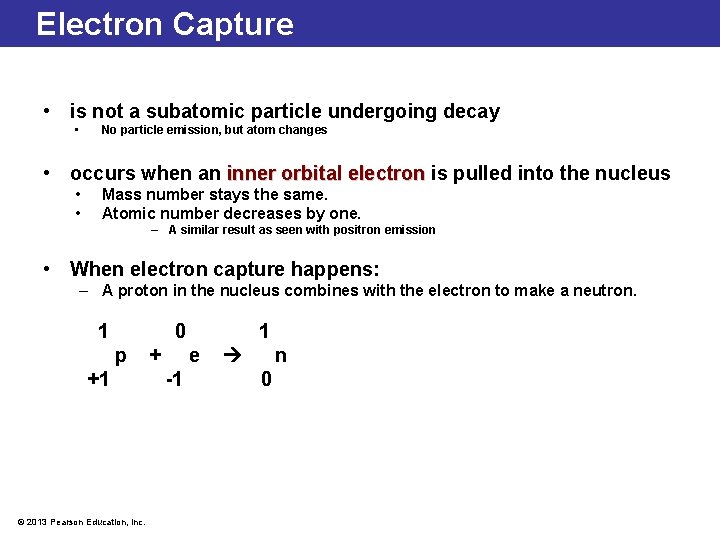
Electron Capture • is not a subatomic particle undergoing decay • No particle emission, but atom changes • occurs when an inner orbital electron is pulled into the nucleus • • Mass number stays the same. Atomic number decreases by one. – A similar result as seen with positron emission • When electron capture happens: – A proton in the nucleus combines with the electron to make a neutron. 1 0 p +1 © 2013 Pearson Education, Inc. + 1 e -1 n 0
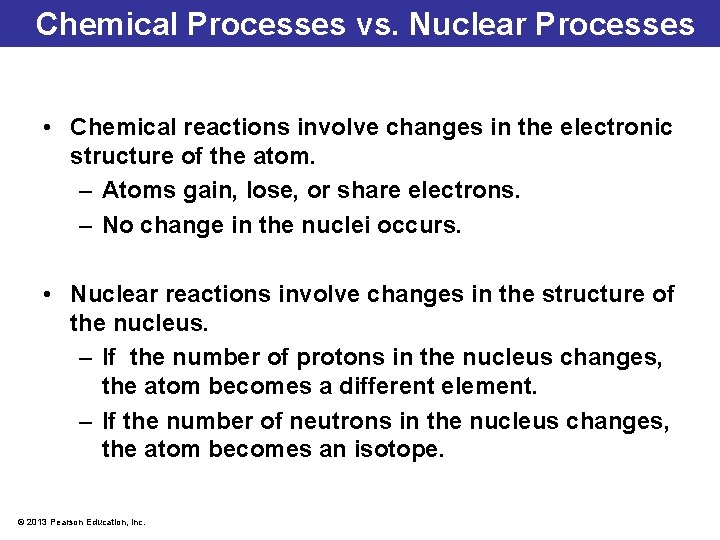
Chemical Processes vs. Nuclear Processes • Chemical reactions involve changes in the electronic structure of the atom. – Atoms gain, lose, or share electrons. – No change in the nuclei occurs. • Nuclear reactions involve changes in the structure of the nucleus. – If the number of protons in the nucleus changes, the atom becomes a different element. – If the number of neutrons in the nucleus changes, the atom becomes an isotope. © 2013 Pearson Education, Inc.
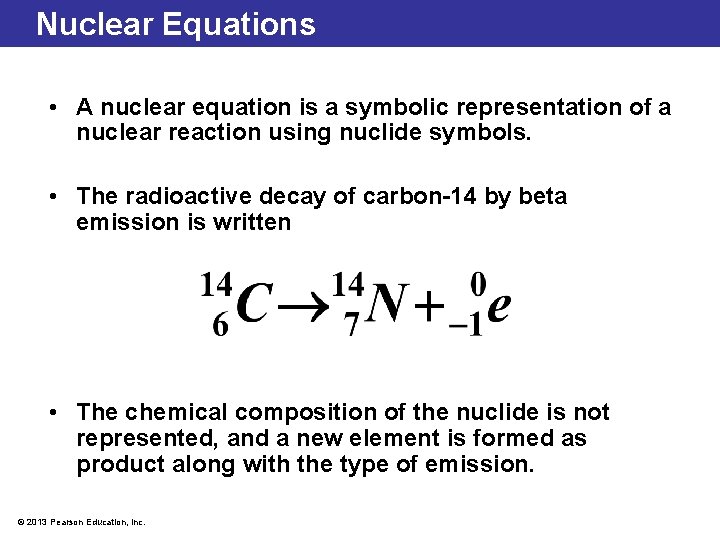
Nuclear Equations • A nuclear equation is a symbolic representation of a nuclear reaction using nuclide symbols. • The radioactive decay of carbon-14 by beta emission is written • The chemical composition of the nuclide is not represented, and a new element is formed as product along with the type of emission. © 2013 Pearson Education, Inc.
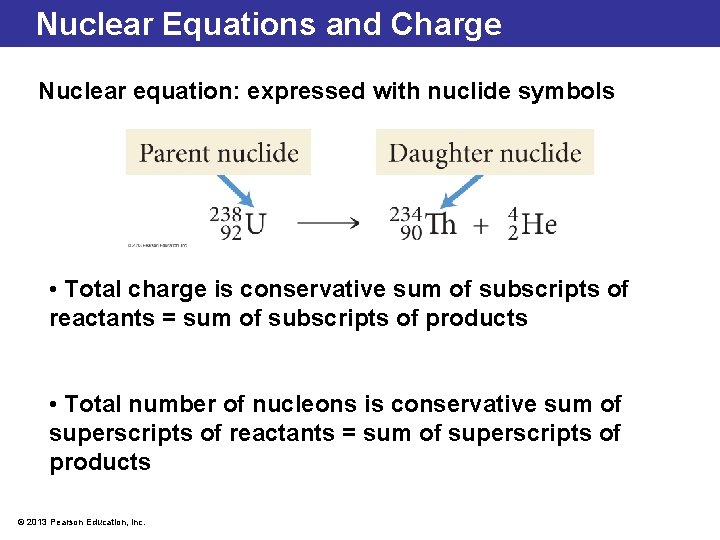
Nuclear Equations and Charge Nuclear equation: expressed with nuclide symbols • Total charge is conservative sum of subscripts of reactants = sum of subscripts of products • Total number of nucleons is conservative sum of superscripts of reactants = sum of superscripts of products © 2013 Pearson Education, Inc.
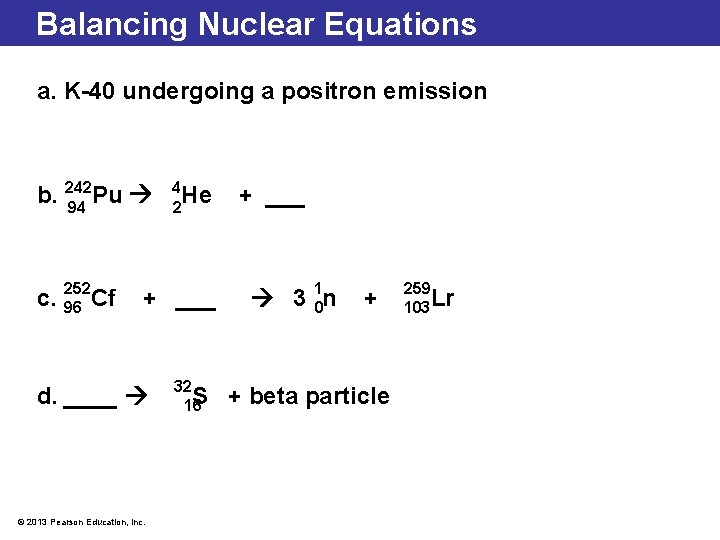
Balancing Nuclear Equations a. K-40 undergoing a positron emission b. 242 Pu 94 252 c. 96 Cf 4 He 2 + ___ d. ____ © 2013 Pearson Education, Inc. 32 S 16 + ___ 3 10 n + + beta particle 259 103 Lr
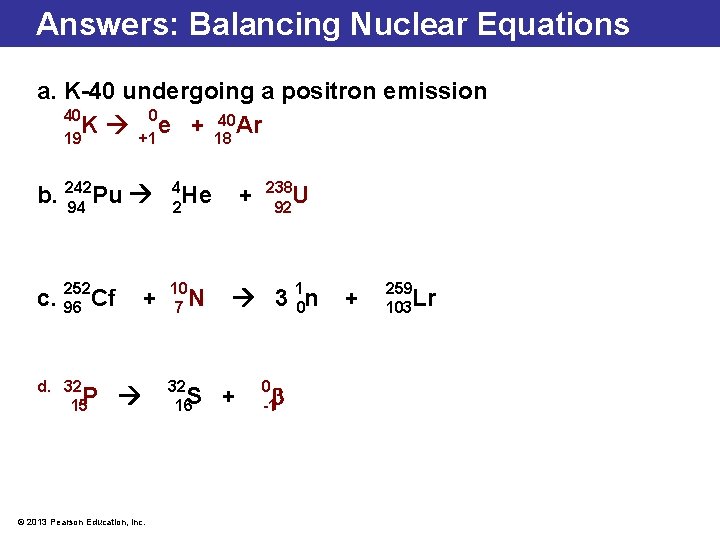
Answers: Balancing Nuclear Equations a. K-40 undergoing a positron emission 40 0 40 Ar K e + 19 +1 18 b. 242 Pu 94 4 He 2 252 c. 96 Cf 10 7 N d. 32 15 P + © 2013 Pearson Education, Inc. 32 16 S + 238 U 92 3 10 n + 0 -1 + 259 103 Lr
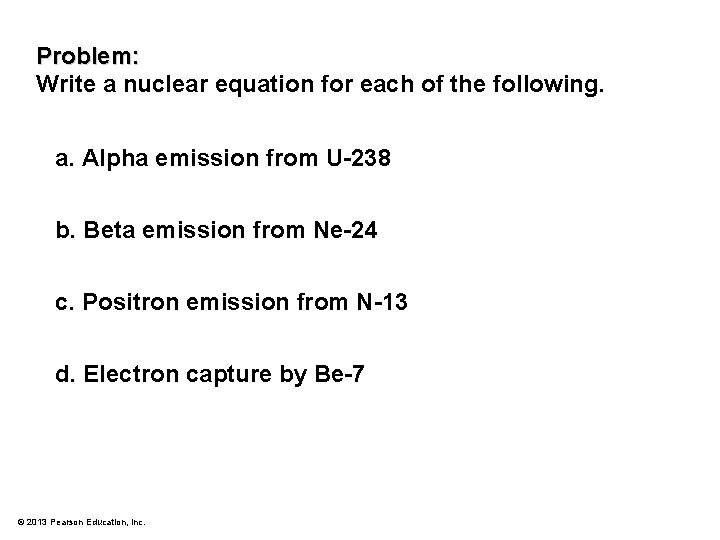
Problem: Write a nuclear equation for each of the following. a. Alpha emission from U-238 b. Beta emission from Ne-24 c. Positron emission from N-13 d. Electron capture by Be-7 © 2013 Pearson Education, Inc.
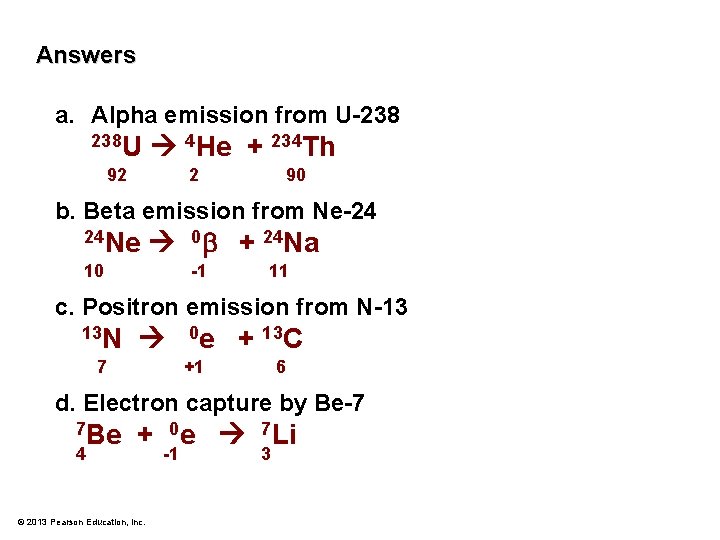
Answers a. Alpha emission from U-238 238 U 4 He + 234 Th 92 2 90 b. Beta emission from Ne-24 24 Ne 0 + 24 Na 10 -1 11 c. Positron emission from N-13 13 N 7 0 e + 13 C +1 6 d. Electron capture by Be-7 7 Be 4 + 0 e 7 Li © 2013 Pearson Education, Inc. -1 3
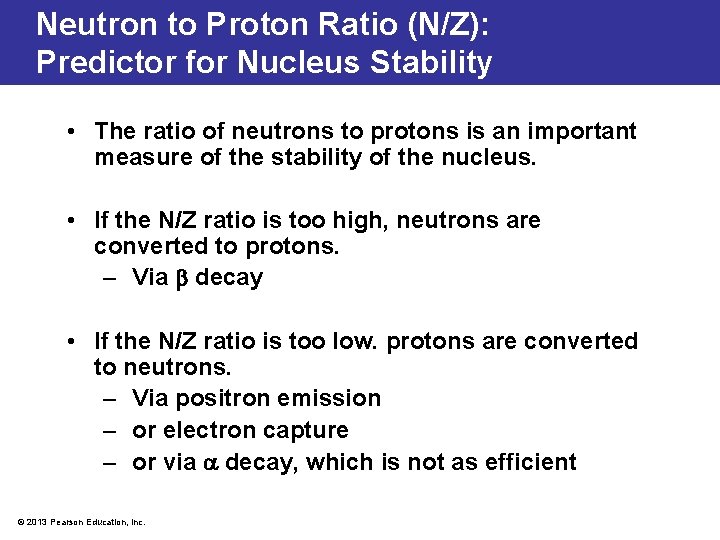
Neutron to Proton Ratio (N/Z): Predictor for Nucleus Stability • The ratio of neutrons to protons is an important measure of the stability of the nucleus. • If the N/Z ratio is too high, neutrons are converted to protons. – Via decay • If the N/Z ratio is too low. protons are converted to neutrons. – Via positron emission – or electron capture – or via decay, which is not as efficient © 2013 Pearson Education, Inc.
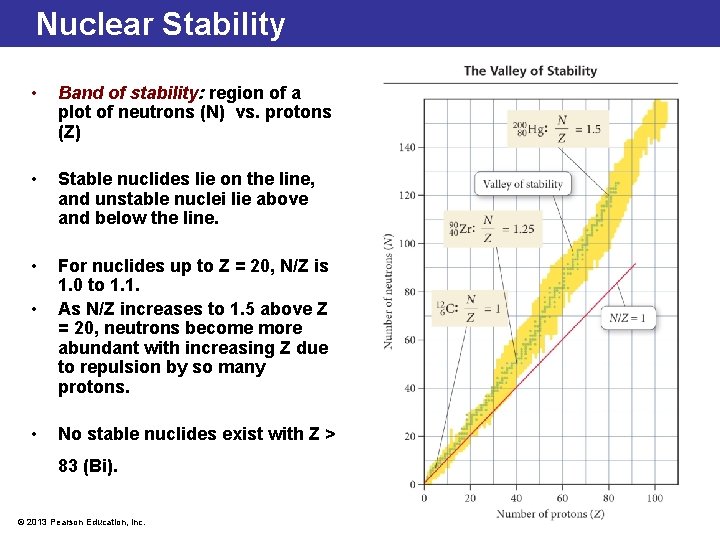
Nuclear Stability • Band of stability: region of a plot of neutrons (N) vs. protons (Z) • Stable nuclides lie on the line, and unstable nuclei lie above and below the line. • For nuclides up to Z = 20, N/Z is 1. 0 to 1. 1. As N/Z increases to 1. 5 above Z = 20, neutrons become more abundant with increasing Z due to repulsion by so many protons. • • No stable nuclides exist with Z > 83 (Bi). © 2013 Pearson Education, Inc.
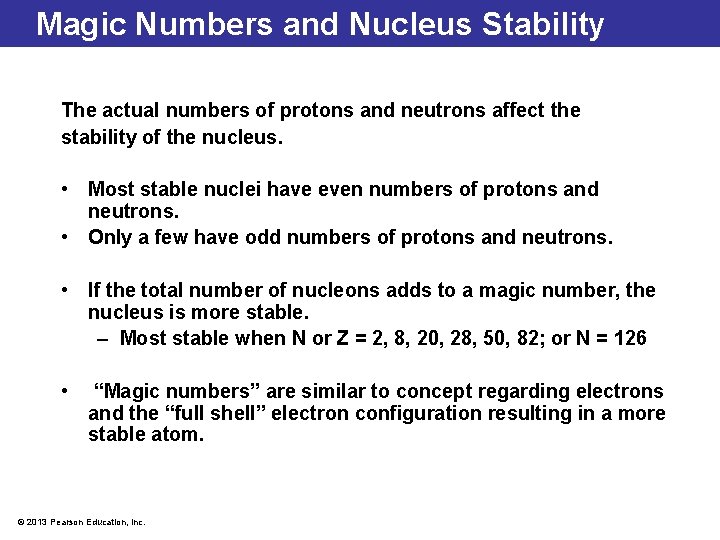
Magic Numbers and Nucleus Stability The actual numbers of protons and neutrons affect the stability of the nucleus. • Most stable nuclei have even numbers of protons and neutrons. • Only a few have odd numbers of protons and neutrons. • If the total number of nucleons adds to a magic number, the nucleus is more stable. – Most stable when N or Z = 2, 8, 20, 28, 50, 82; or N = 126 • “Magic numbers” are similar to concept regarding electrons and the “full shell” electron configuration resulting in a more stable atom. © 2013 Pearson Education, Inc.
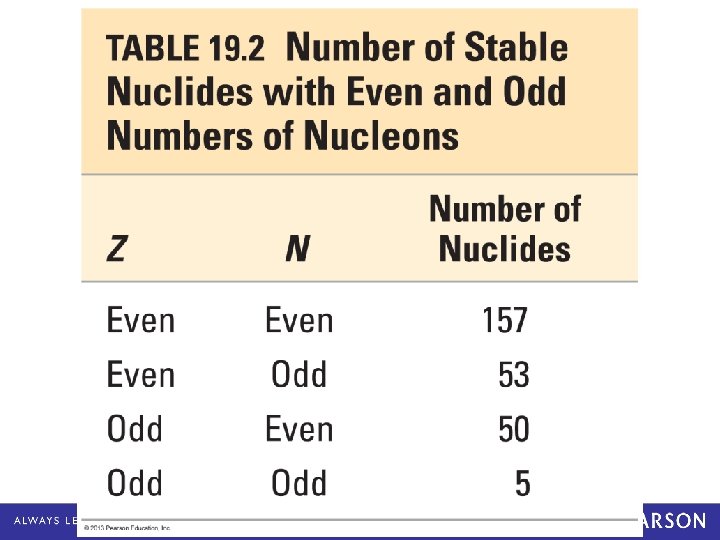
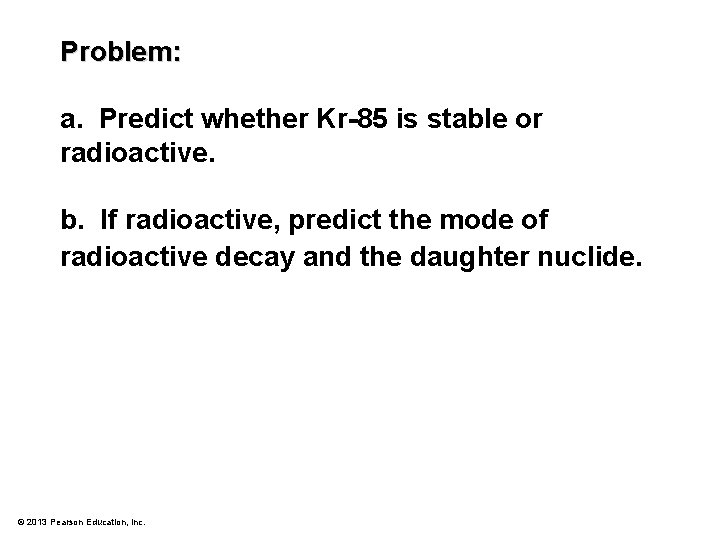
Problem: a. Predict whether Kr-85 is stable or radioactive. b. If radioactive, predict the mode of radioactive decay and the daughter nuclide. . © 2013 Pearson Education, Inc.
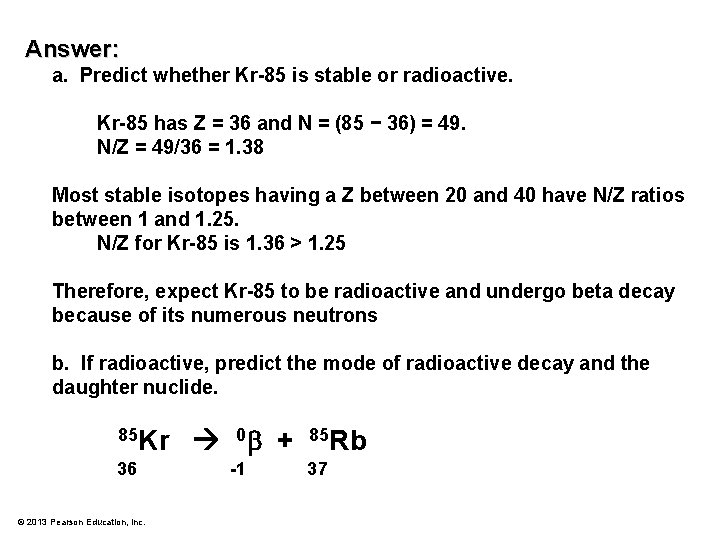
Answer: a. Predict whether Kr-85 is stable or radioactive. Kr-85 has Z = 36 and N = (85 − 36) = 49. N/Z = 49/36 = 1. 38 Most stable isotopes having a Z between 20 and 40 have N/Z ratios between 1 and 1. 25. N/Z for Kr-85 is 1. 36 > 1. 25 Therefore, expect Kr-85 to be radioactive and undergo beta decay because of its numerous neutrons b. If radioactive, predict the mode of radioactive decay and the daughter nuclide. 85 Kr 36 © 2013 Pearson Education, Inc. 0 + -1 85 Rb 37
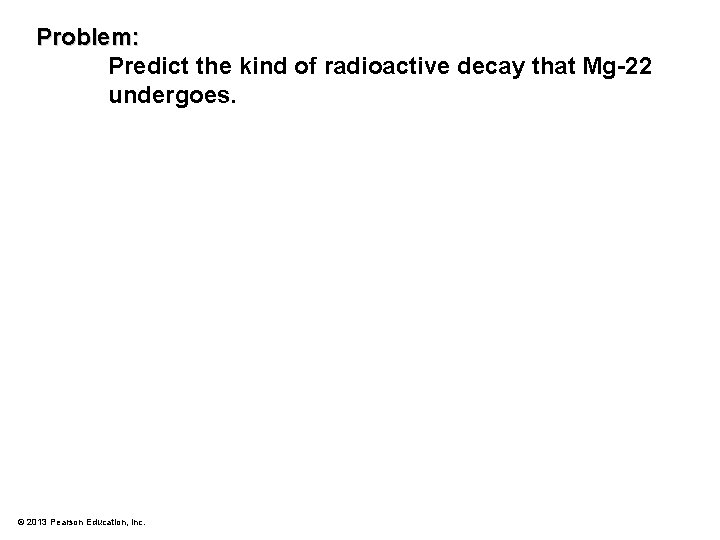
Problem: Predict the kind of radioactive decay that Mg-22 undergoes. © 2013 Pearson Education, Inc.
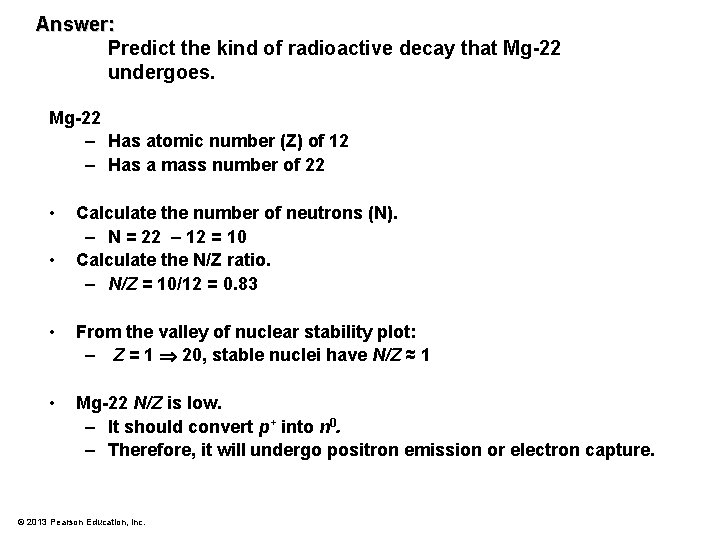
Answer: Predict the kind of radioactive decay that Mg-22 undergoes. Mg-22 – Has atomic number (Z) of 12 – Has a mass number of 22 • • Calculate the number of neutrons (N). – N = 22 – 12 = 10 Calculate the N/Z ratio. – N/Z = 10/12 = 0. 83 • From the valley of nuclear stability plot: – Z = 1 20, stable nuclei have N/Z ≈ 1 • Mg-22 N/Z is low. – It should convert p+ into n 0. – Therefore, it will undergo positron emission or electron capture. © 2013 Pearson Education, Inc.
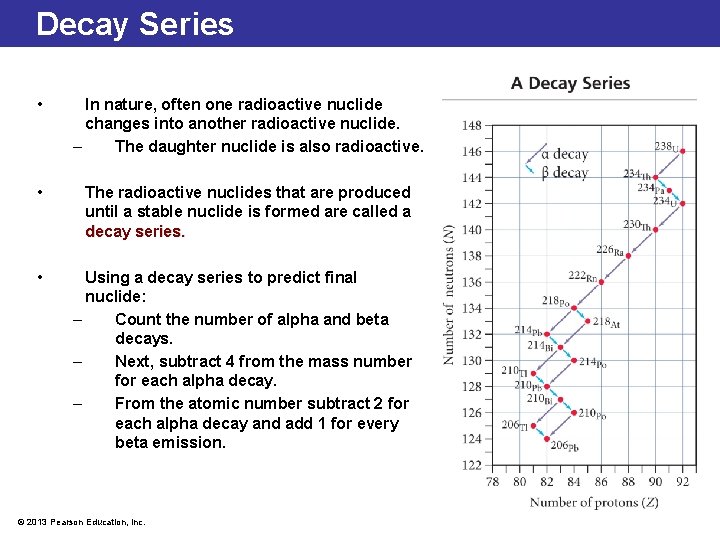
Decay Series • In nature, often one radioactive nuclide changes into another radioactive nuclide. – The daughter nuclide is also radioactive. • The radioactive nuclides that are produced until a stable nuclide is formed are called a decay series. • Using a decay series to predict final nuclide: – Count the number of alpha and beta decays. – Next, subtract 4 from the mass number for each alpha decay. – From the atomic number subtract 2 for each alpha decay and add 1 for every beta emission. © 2013 Pearson Education, Inc.
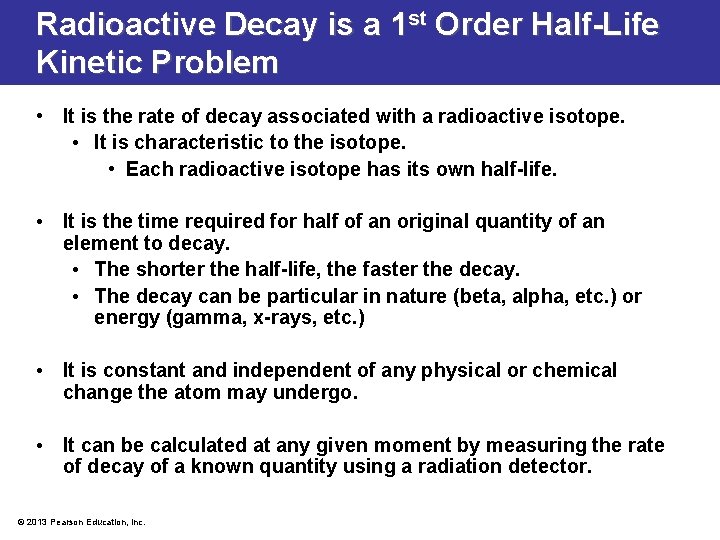
Radioactive Decay is a 1 st Order Half-Life Kinetic Problem • It is the rate of decay associated with a radioactive isotope. • It is characteristic to the isotope. • Each radioactive isotope has its own half-life. • It is the time required for half of an original quantity of an element to decay. • The shorter the half-life, the faster the decay. • The decay can be particular in nature (beta, alpha, etc. ) or energy (gamma, x-rays, etc. ) • It is constant and independent of any physical or chemical change the atom may undergo. • It can be calculated at any given moment by measuring the rate of decay of a known quantity using a radiation detector. © 2013 Pearson Education, Inc.
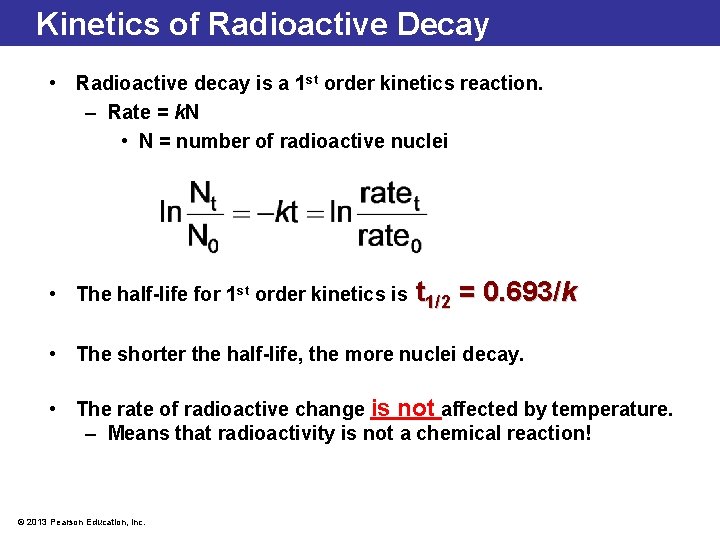
Kinetics of Radioactive Decay • Radioactive decay is a 1 st order kinetics reaction. – Rate = k. N • N = number of radioactive nuclei • The half-life for 1 st order kinetics is t 1/2 = 0. 693/k • The shorter the half-life, the more nuclei decay. • The rate of radioactive change is not affected by temperature. – Means that radioactivity is not a chemical reaction! © 2013 Pearson Education, Inc.
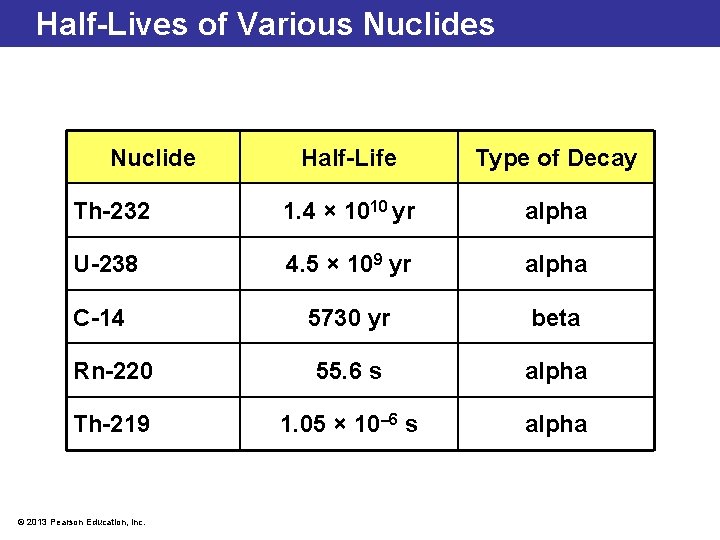
Half-Lives of Various Nuclide Half-Life Type of Decay Th-232 1. 4 × 1010 yr alpha U-238 4. 5 × 109 yr alpha C-14 5730 yr beta Rn-220 55. 6 s alpha Th-219 1. 05 × 10– 6 s alpha © 2013 Pearson Education, Inc.
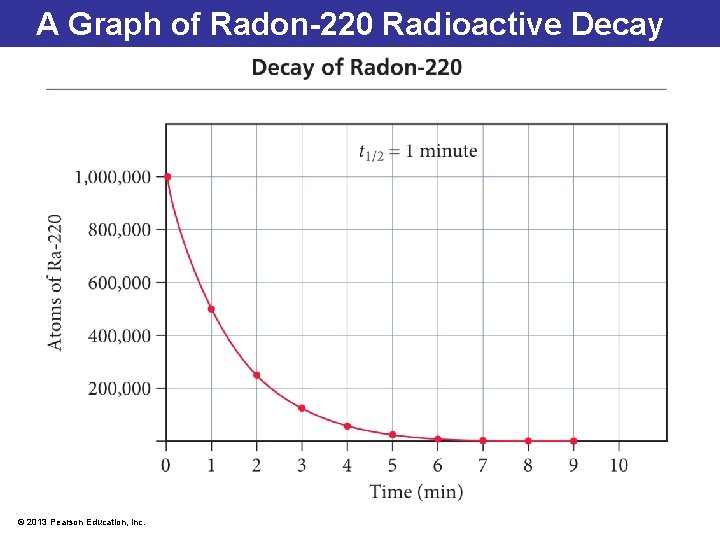
A Graph of Radon-220 Radioactive Decay © 2013 Pearson Education, Inc.
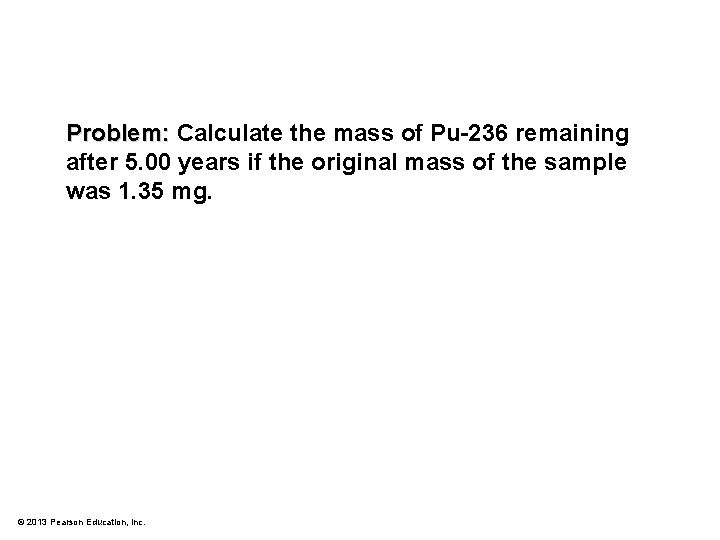
Problem: Calculate the mass of Pu-236 remaining after 5. 00 years if the original mass of the sample was 1. 35 mg. © 2013 Pearson Education, Inc.
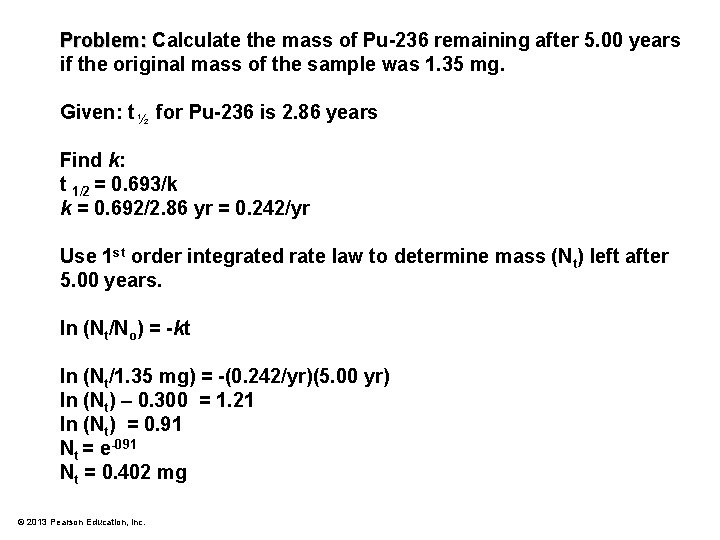
Problem: Calculate the mass of Pu-236 remaining after 5. 00 years if the original mass of the sample was 1. 35 mg. Given: t ½ for Pu-236 is 2. 86 years Find k: t 1/2 = 0. 693/k k = 0. 692/2. 86 yr = 0. 242/yr Use 1 st order integrated rate law to determine mass (Nt) left after 5. 00 years. ln (Nt/No) = -kt ln (Nt/1. 35 mg) = -(0. 242/yr)(5. 00 yr) ln (Nt) – 0. 300 = 1. 21 ln (Nt) = 0. 91 Nt = e-091 Nt = 0. 402 mg © 2013 Pearson Education, Inc.
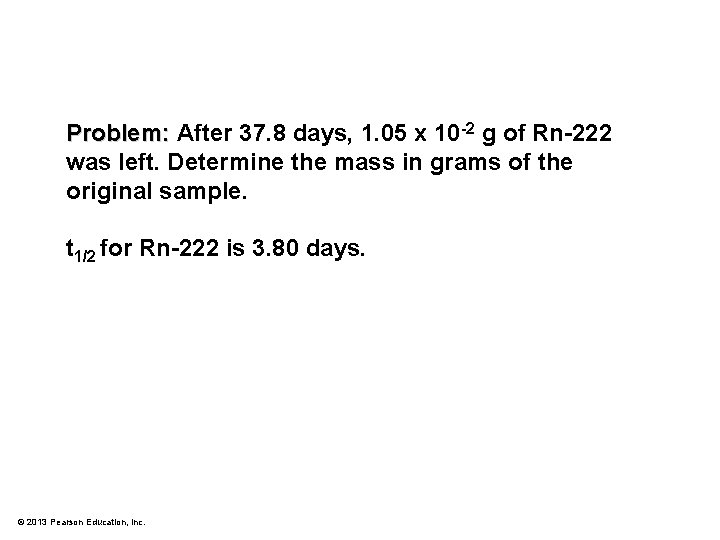
Problem: After 37. 8 days, 1. 05 x 10 -2 g of Rn-222 was left. Determine the mass in grams of the original sample. t 1/2 for Rn-222 is 3. 80 days. © 2013 Pearson Education, Inc.
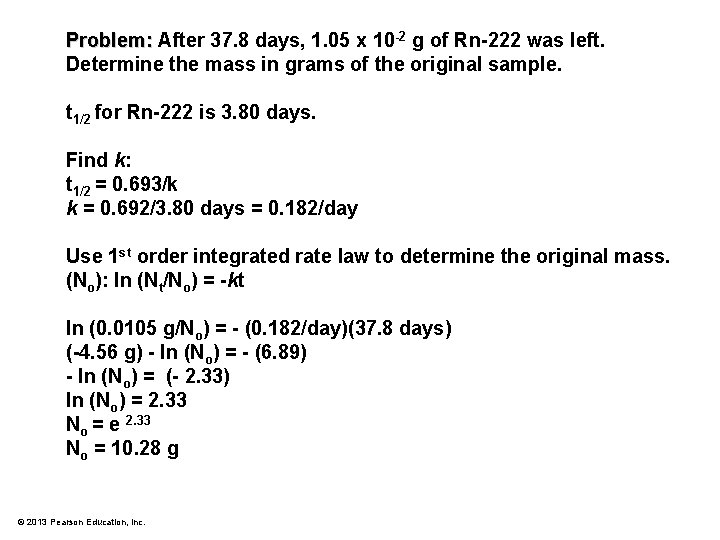
Problem: After 37. 8 days, 1. 05 x 10 -2 g of Rn-222 was left. Determine the mass in grams of the original sample. t 1/2 for Rn-222 is 3. 80 days. Find k: t 1/2 = 0. 693/k k = 0. 692/3. 80 days = 0. 182/day Use 1 st order integrated rate law to determine the original mass. (No): ln (Nt/No) = -kt ln (0. 0105 g/No) = - (0. 182/day)(37. 8 days) (-4. 56 g) - ln (No) = - (6. 89) - ln (No) = (- 2. 33) ln (No) = 2. 33 No = e 2. 33 No = 10. 28 g © 2013 Pearson Education, Inc.
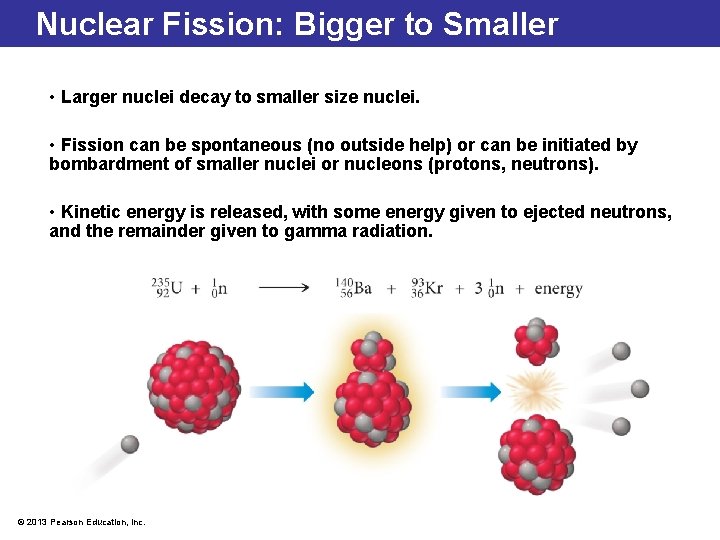
Nuclear Fission: Bigger to Smaller • Larger nuclei decay to smaller size nuclei. • Fission can be spontaneous (no outside help) or can be initiated by bombardment of smaller nuclei or nucleons (protons, neutrons). • Kinetic energy is released, with some energy given to ejected neutrons, and the remainder given to gamma radiation. © 2013 Pearson Education, Inc.
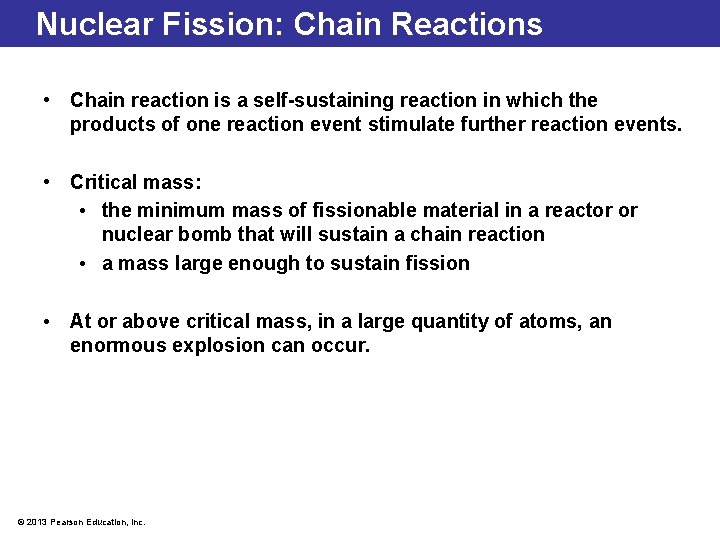
Nuclear Fission: Chain Reactions • Chain reaction is a self-sustaining reaction in which the products of one reaction event stimulate further reaction events. • Critical mass: • the minimum mass of fissionable material in a reactor or nuclear bomb that will sustain a chain reaction • a mass large enough to sustain fission • At or above critical mass, in a large quantity of atoms, an enormous explosion can occur. © 2013 Pearson Education, Inc.
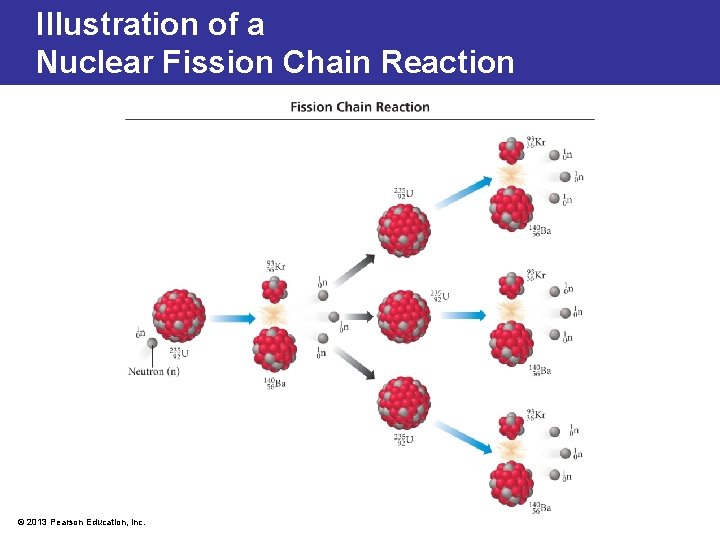
Illustration of a Nuclear Fission Chain Reaction © 2013 Pearson Education, Inc.
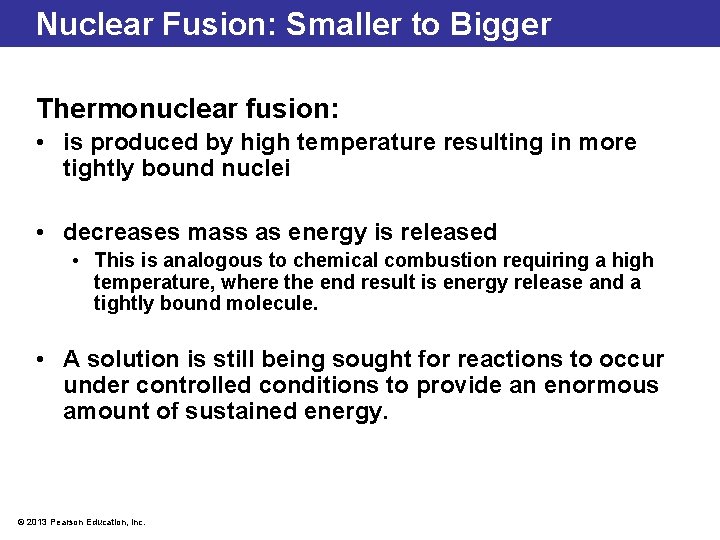
Nuclear Fusion: Smaller to Bigger Thermonuclear fusion: • is produced by high temperature resulting in more tightly bound nuclei • decreases mass as energy is released • This is analogous to chemical combustion requiring a high temperature, where the end result is energy release and a tightly bound molecule. • A solution is still being sought for reactions to occur under controlled conditions to provide an enormous amount of sustained energy. © 2013 Pearson Education, Inc.
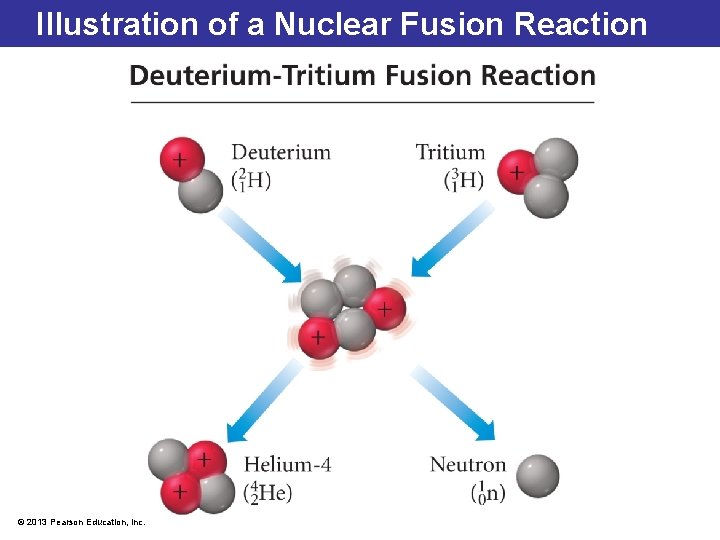
Illustration of a Nuclear Fusion Reaction © 2013 Pearson Education, Inc.
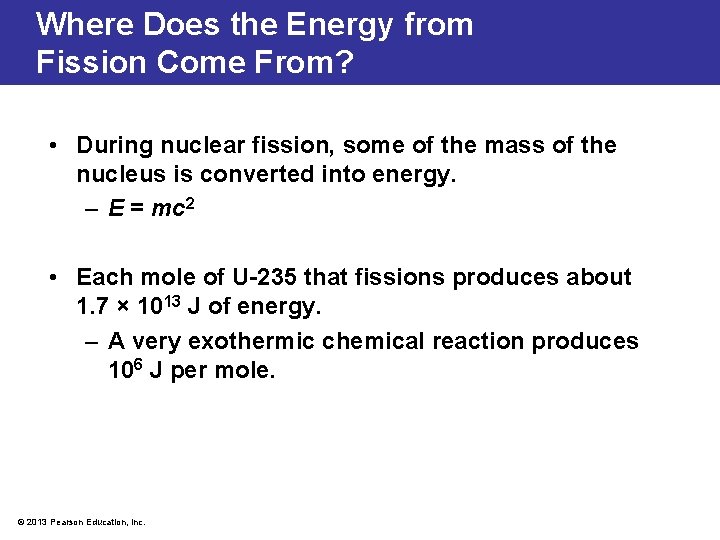
Where Does the Energy from Fission Come From? • During nuclear fission, some of the mass of the nucleus is converted into energy. – E = mc 2 • Each mole of U-235 that fissions produces about 1. 7 × 1013 J of energy. – A very exothermic chemical reaction produces 106 J per mole. © 2013 Pearson Education, Inc.
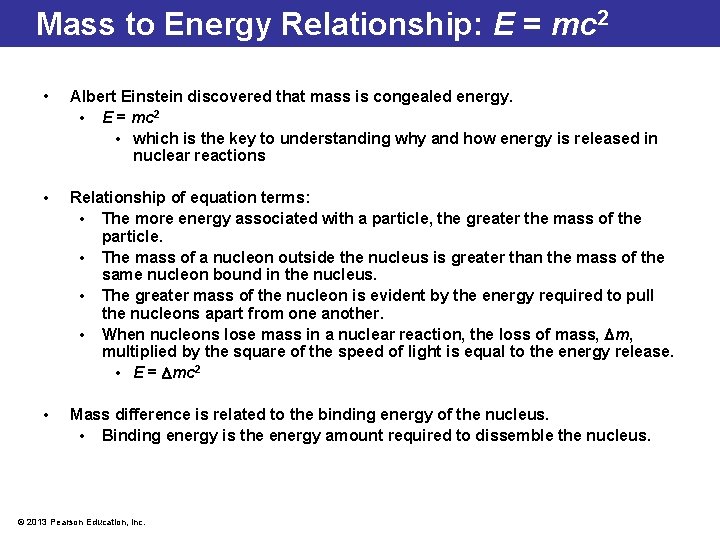
Mass to Energy Relationship: E = mc 2 • Albert Einstein discovered that mass is congealed energy. • E = mc 2 • which is the key to understanding why and how energy is released in nuclear reactions • Relationship of equation terms: • The more energy associated with a particle, the greater the mass of the particle. • The mass of a nucleon outside the nucleus is greater than the mass of the same nucleon bound in the nucleus. • The greater mass of the nucleon is evident by the energy required to pull the nucleons apart from one another. • When nucleons lose mass in a nuclear reaction, the loss of mass, m, multiplied by the square of the speed of light is equal to the energy release. • E = mc 2 • Mass difference is related to the binding energy of the nucleus. • Binding energy is the energy amount required to dissemble the nucleus. © 2013 Pearson Education, Inc.
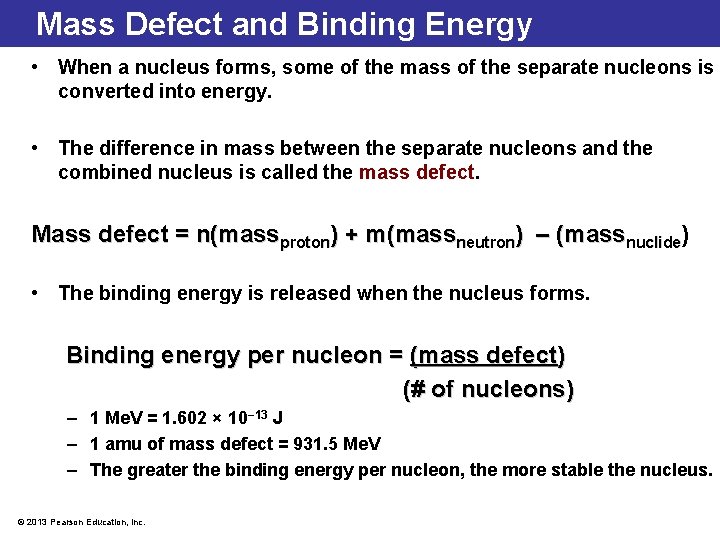
Mass Defect and Binding Energy • When a nucleus forms, some of the mass of the separate nucleons is converted into energy. • The difference in mass between the separate nucleons and the combined nucleus is called the mass defect. Mass defect = n(massproton) + m(massneutron) – (massnuclide) • The binding energy is released when the nucleus forms. Binding energy per nucleon = (mass defect) (# of nucleons) – 1 Me. V = 1. 602 × 10− 13 J – 1 amu of mass defect = 931. 5 Me. V – The greater the binding energy per nucleon, the more stable the nucleus. © 2013 Pearson Education, Inc.
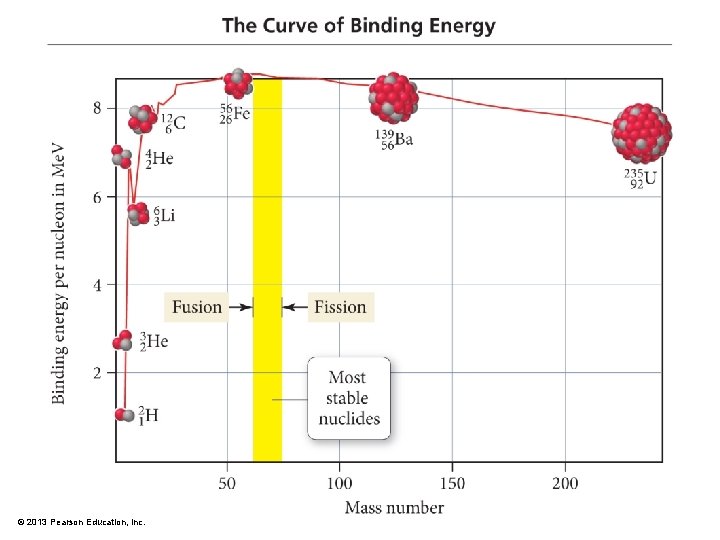
© 2013 Pearson Education, Inc.
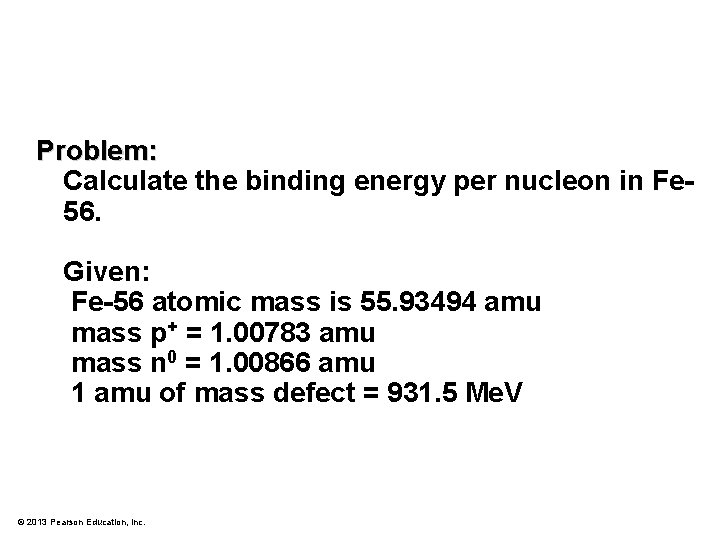
Problem: Calculate the binding energy per nucleon in Fe 56. Given: Fe-56 atomic mass is 55. 93494 amu mass p+ = 1. 00783 amu mass n 0 = 1. 00866 amu 1 amu of mass defect = 931. 5 Me. V © 2013 Pearson Education, Inc.
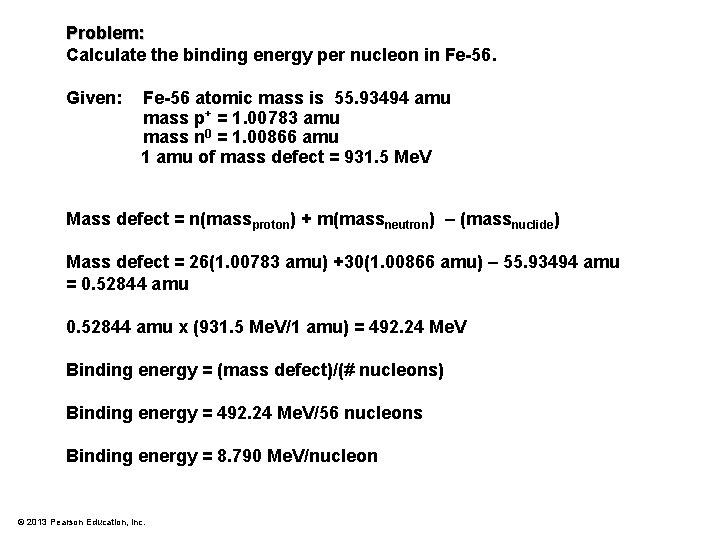
Problem: Calculate the binding energy per nucleon in Fe-56. Given: Fe-56 atomic mass is 55. 93494 amu mass p+ = 1. 00783 amu mass n 0 = 1. 00866 amu 1 amu of mass defect = 931. 5 Me. V Mass defect = n(massproton) + m(massneutron) – (massnuclide) Mass defect = 26(1. 00783 amu) +30(1. 00866 amu) – 55. 93494 amu = 0. 52844 amu x (931. 5 Me. V/1 amu) = 492. 24 Me. V Binding energy = (mass defect)/(# nucleons) Binding energy = 492. 24 Me. V/56 nucleons Binding energy = 8. 790 Me. V/nucleon © 2013 Pearson Education, Inc.
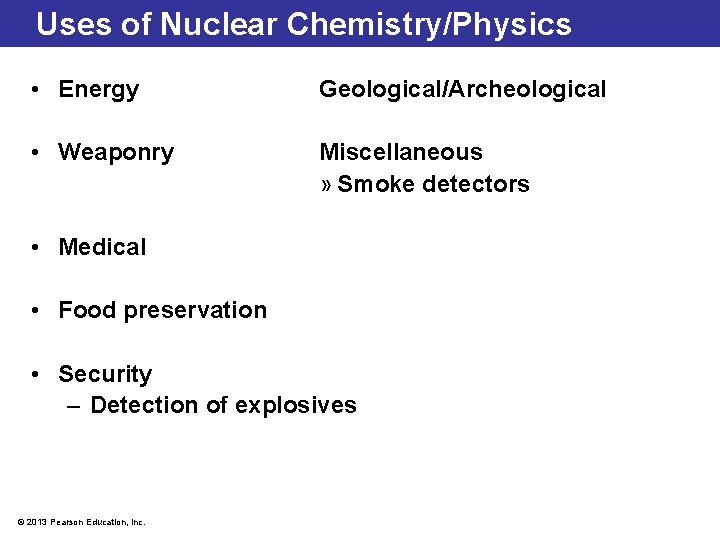
Uses of Nuclear Chemistry/Physics • Energy Geological/Archeological • Weaponry Miscellaneous » Smoke detectors • Medical • Food preservation • Security – Detection of explosives © 2013 Pearson Education, Inc.
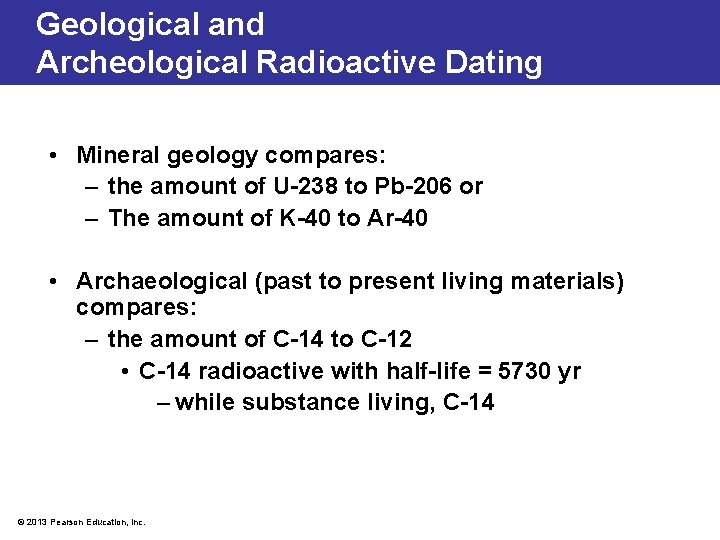
Geological and Archeological Radioactive Dating • Mineral geology compares: – the amount of U-238 to Pb-206 or – The amount of K-40 to Ar-40 • Archaeological (past to present living materials) compares: – the amount of C-14 to C-12 • C-14 radioactive with half-life = 5730 yr – while substance living, C-14 © 2013 Pearson Education, Inc.
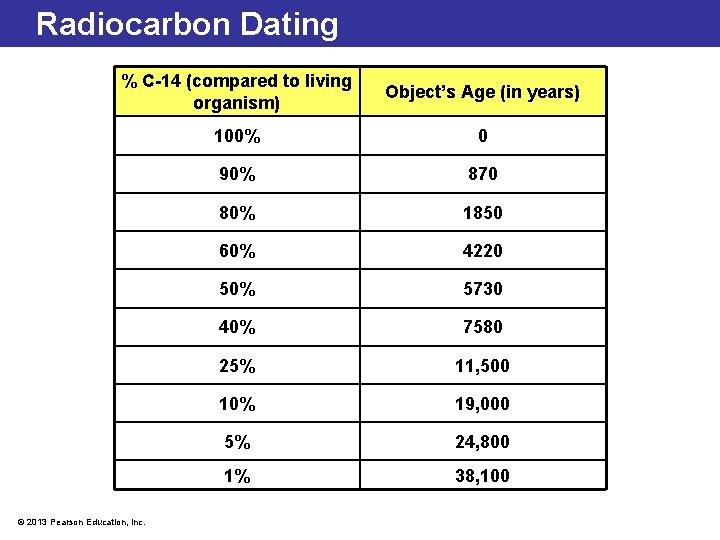
Radiocarbon Dating % C-14 (compared to living organism) Object’s Age (in years) 100% 0 90% 870 80% 1850 60% 4220 50% 5730 40% 7580 25% 11, 500 10% 19, 000 5% 24, 800 1% 38, 100 © 2013 Pearson Education, Inc.
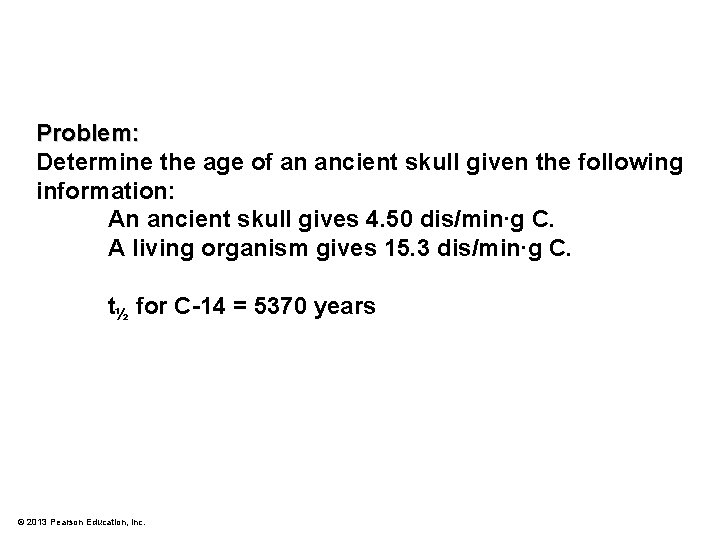
Problem: Determine the age of an ancient skull given the following information: An ancient skull gives 4. 50 dis/min∙g C. A living organism gives 15. 3 dis/min∙g C. t½ for C-14 = 5370 years © 2013 Pearson Education, Inc.
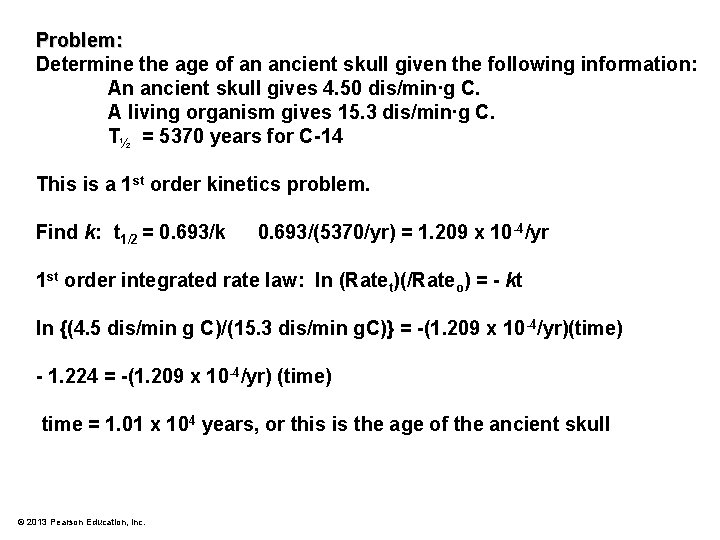
Problem: Determine the age of an ancient skull given the following information: An ancient skull gives 4. 50 dis/min∙g C. A living organism gives 15. 3 dis/min∙g C. T½ = 5370 years for C-14 This is a 1 st order kinetics problem. Find k: t 1/2 = 0. 693/k 0. 693/(5370/yr) = 1. 209 x 10 -4/yr 1 st order integrated rate law: ln (Ratet)(/Rateo) = - kt ln {(4. 5 dis/min g C)/(15. 3 dis/min g. C)} = -(1. 209 x 10 -4/yr)(time) - 1. 224 = -(1. 209 x 10 -4/yr) (time) time = 1. 01 x 104 years, or this is the age of the ancient skull © 2013 Pearson Education, Inc.
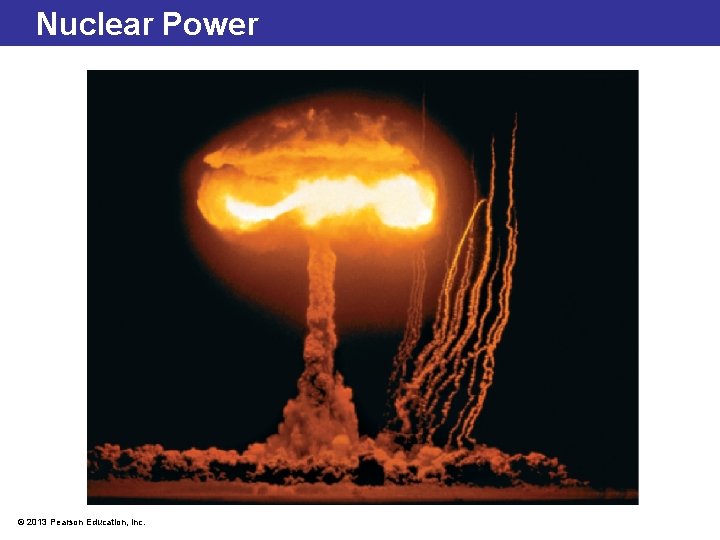
Nuclear Power © 2013 Pearson Education, Inc.
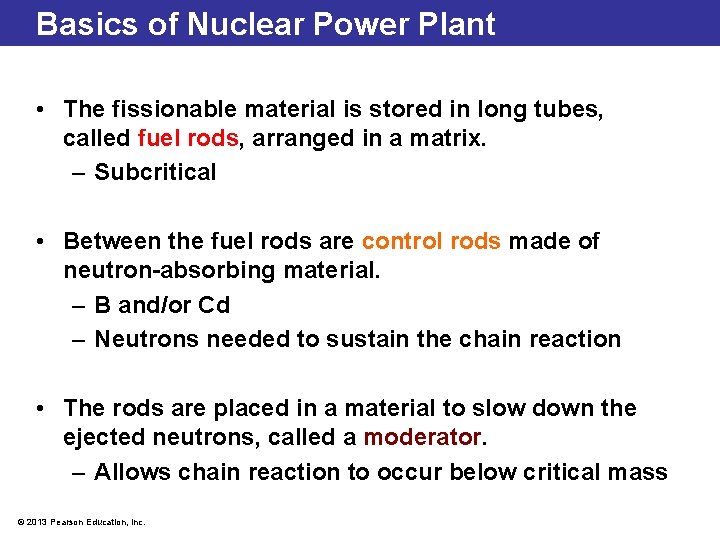
Basics of Nuclear Power Plant • The fissionable material is stored in long tubes, called fuel rods, arranged in a matrix. – Subcritical • Between the fuel rods are control rods made of neutron-absorbing material. – B and/or Cd – Neutrons needed to sustain the chain reaction • The rods are placed in a material to slow down the ejected neutrons, called a moderator. – Allows chain reaction to occur below critical mass © 2013 Pearson Education, Inc.
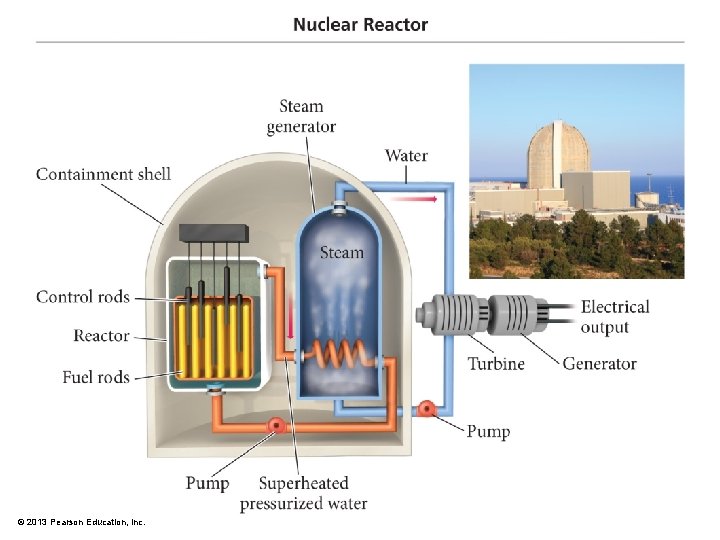
© 2013 Pearson Education, Inc.
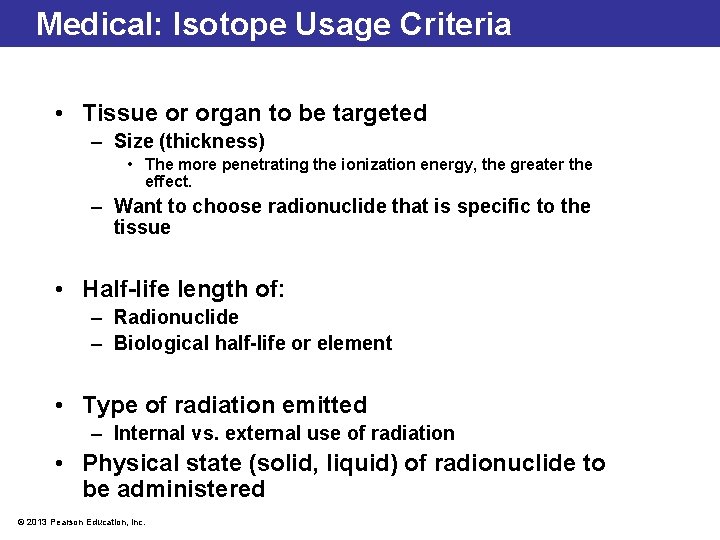
Medical: Isotope Usage Criteria • Tissue or organ to be targeted – Size (thickness) • The more penetrating the ionization energy, the greater the effect. – Want to choose radionuclide that is specific to the tissue • Half-life length of: – Radionuclide – Biological half-life or element • Type of radiation emitted – Internal vs. external use of radiation • Physical state (solid, liquid) of radionuclide to be administered © 2013 Pearson Education, Inc.
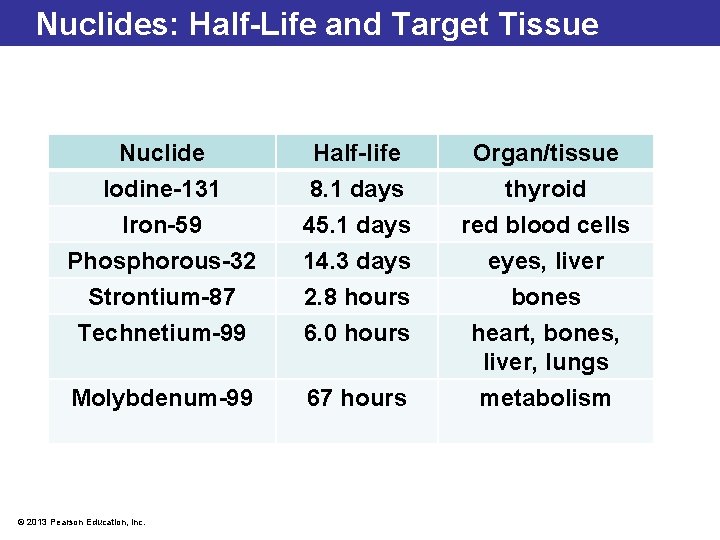
Nuclides: Half-Life and Target Tissue Nuclide Half-life Organ/tissue Iodine-131 8. 1 days thyroid Iron-59 45. 1 days red blood cells Phosphorous-32 14. 3 days eyes, liver Strontium-87 2. 8 hours bones Technetium-99 6. 0 hours heart, bones, liver, lungs Molybdenum-99 67 hours metabolism © 2013 Pearson Education, Inc.
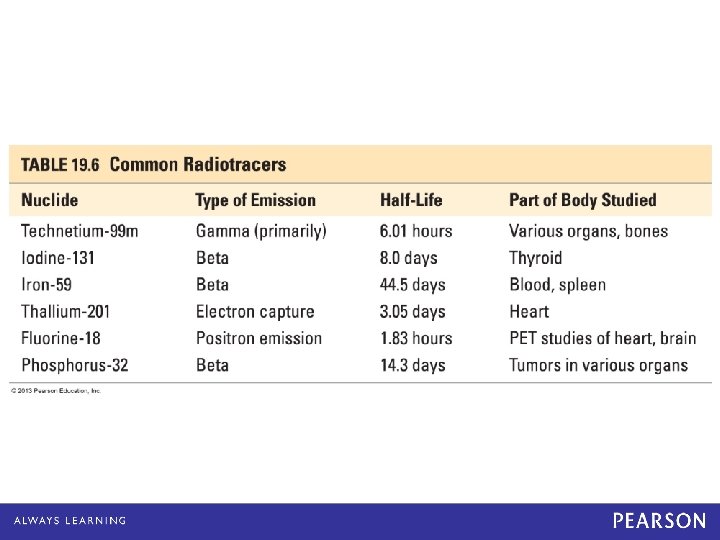
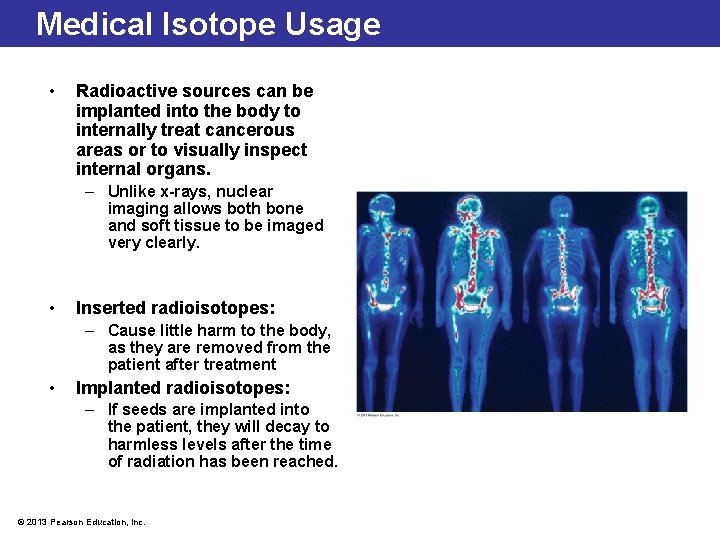
Medical Isotope Usage • Radioactive sources can be implanted into the body to internally treat cancerous areas or to visually inspect internal organs. – Unlike x-rays, nuclear imaging allows both bone and soft tissue to be imaged very clearly. • Inserted radioisotopes: – Cause little harm to the body, as they are removed from the patient after treatment • Implanted radioisotopes: – If seeds are implanted into the patient, they will decay to harmless levels after the time of radiation has been reached. © 2013 Pearson Education, Inc.
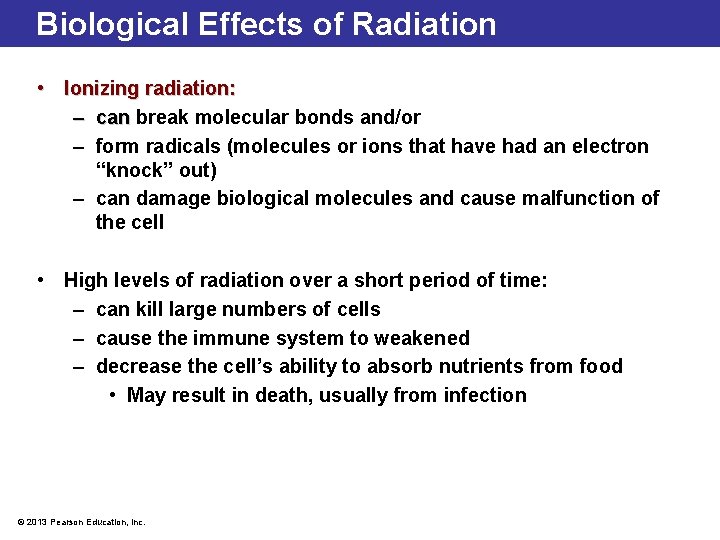
Biological Effects of Radiation • Ionizing radiation: – can break molecular bonds and/or – form radicals (molecules or ions that have had an electron “knock” out) – can damage biological molecules and cause malfunction of the cell • High levels of radiation over a short period of time: – can kill large numbers of cells – cause the immune system to weakened – decrease the cell’s ability to absorb nutrients from food • May result in death, usually from infection © 2013 Pearson Education, Inc.
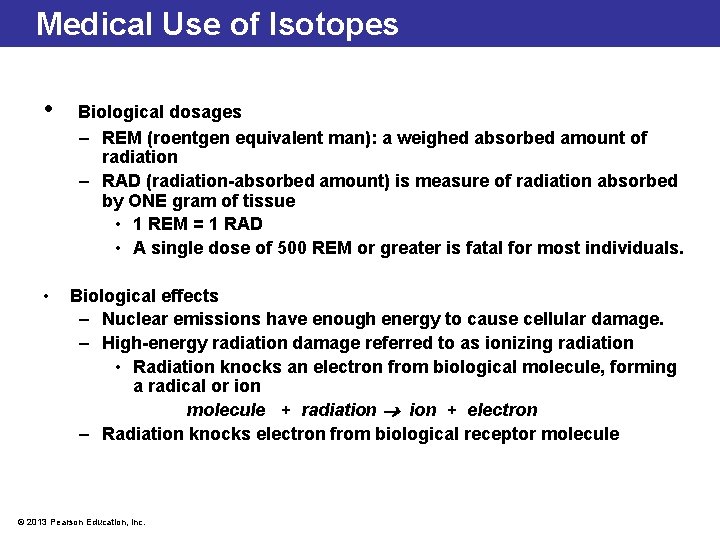
Medical Use of Isotopes • • Biological dosages – REM (roentgen equivalent man): a weighed absorbed amount of radiation – RAD (radiation-absorbed amount) is measure of radiation absorbed by ONE gram of tissue • 1 REM = 1 RAD • A single dose of 500 REM or greater is fatal for most individuals. Biological effects – Nuclear emissions have enough energy to cause cellular damage. – High-energy radiation damage referred to as ionizing radiation • Radiation knocks an electron from biological molecule, forming a radical or ion molecule + radiation + electron – Radiation knocks electron from biological receptor molecule © 2013 Pearson Education, Inc.
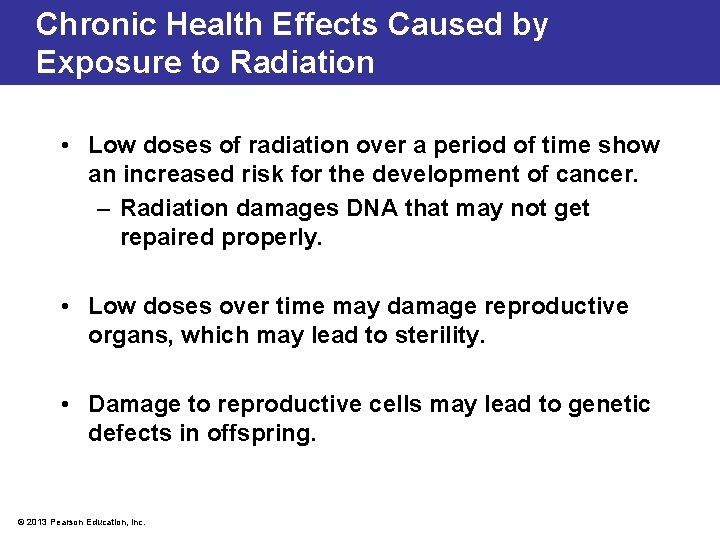
Chronic Health Effects Caused by Exposure to Radiation • Low doses of radiation over a period of time show an increased risk for the development of cancer. – Radiation damages DNA that may not get repaired properly. • Low doses over time may damage reproductive organs, which may lead to sterility. • Damage to reproductive cells may lead to genetic defects in offspring. © 2013 Pearson Education, Inc.
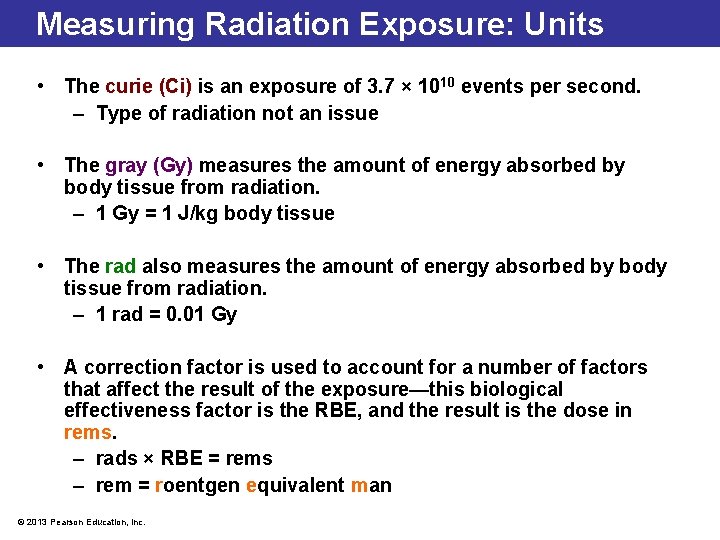
Measuring Radiation Exposure: Units • The curie (Ci) is an exposure of 3. 7 × 1010 events per second. – Type of radiation not an issue • The gray (Gy) measures the amount of energy absorbed by body tissue from radiation. – 1 Gy = 1 J/kg body tissue • The rad also measures the amount of energy absorbed by body tissue from radiation. – 1 rad = 0. 01 Gy • A correction factor is used to account for a number of factors that affect the result of the exposure—this biological effectiveness factor is the RBE, and the result is the dose in rems. – rads × RBE = rems – rem = roentgen equivalent man © 2013 Pearson Education, Inc.
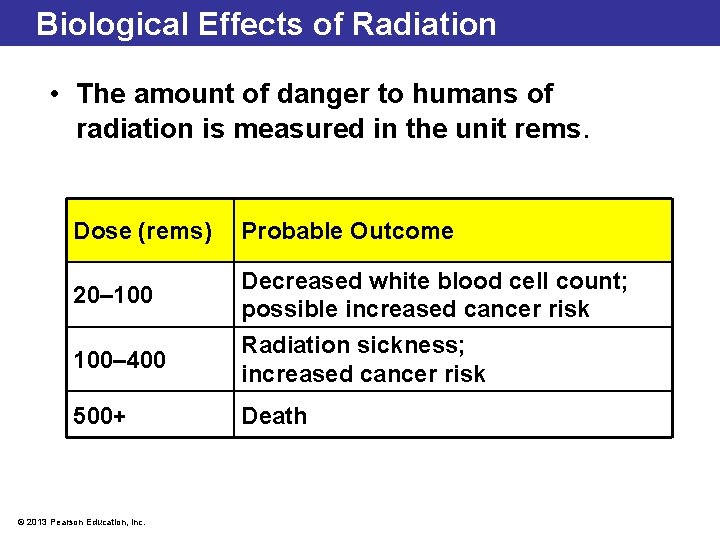
Biological Effects of Radiation • The amount of danger to humans of radiation is measured in the unit rems. Dose (rems) Probable Outcome 20– 100 Decreased white blood cell count; possible increased cancer risk 100– 400 Radiation sickness; increased cancer risk 500+ Death © 2013 Pearson Education, Inc.
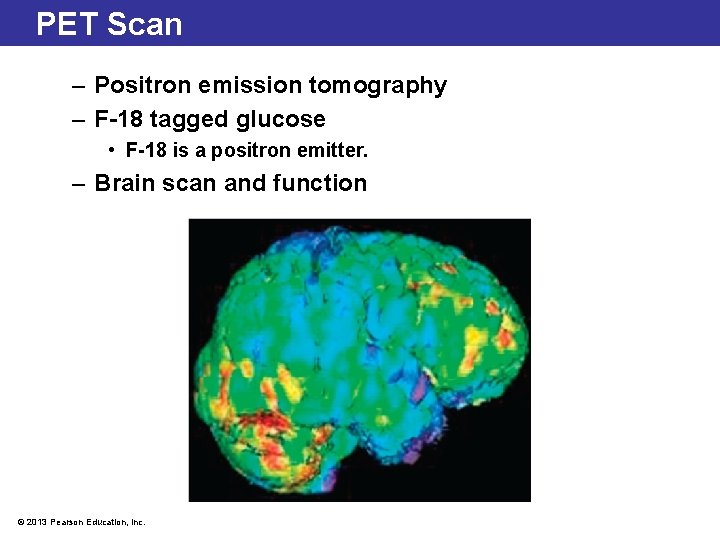
PET Scan – Positron emission tomography – F-18 tagged glucose • F-18 is a positron emitter. – Brain scan and function © 2013 Pearson Education, Inc.
Key terms radioactivity and nuclear reactions
12x12x12x12x12x12x12
Chapter 25 nuclear chemistry answer key
Chapter 21 review nuclear chemistry
Chapter 25 nuclear chemistry
Chapter 25 nuclear chemistry
Chapter 10 nuclear chemistry
Chapter 10 nuclear chemistry
Lesson 15 nuclear quest nuclear reactions
Fisión nuclear vs fision nuclear
Natural and artificial radioactivity
Natural transmutation example
Natural and artificial radioactivity
Datación radiométrica
01:640:244 lecture notes - lecture 15: plat, idah, farad
Becquerel discovery of radioactivity
Who discovered radioactivity
Who discovered radioactivity
Law of radioactive decay
Radioactivity as spontaneous disintegration
Mta ek
Radioactivity
The great unconformity
Natural radioactivity
Environmental radioactivity
Natural radioactivity
Radioactivity phenomenon
Units of radioactivity
Radioactive formula
Defination of radioactivity
Defination of radioactivity
Defination of radioactivity
Gamma emission equation
Chernobyl nuclear disaster webquest
Chemistry
Application of nuclear chemistry
Application of nuclear chemistry
What is nuclear charge in chemistry
Nuclear chemistry review worksheet answer key
25 m/s
Nuclear chemistry
Rectangle real life examples
Nuclear chemistry
Nitrogen-13 decay equation
Irradiated food
Nuclear physics topics for presentation
Advanced inorganic chemistry lecture notes
An introduction to atmospheric physics
Lecture presentation software
Ib chemistry organic chemistry
Inorganic vs organic chemistry
5cm labor
Vertex presentation
Human resource management 15th edition
Intro to human resource management
Human resource management lecture chapter 1
Chemistry matter and change chapter 7
Chemistry matter and change chapter 10 the mole answer key
Chemistry chapter 8 review chemical equations and reactions
Chapter 9 chemical names and formulas answer key
Chemistry matter and change chapter 7
Chemistry matter and change chapter 6
Chemistry matter and change chapter 10
Chemistry matter and change answer key chapter 2
Ap chemistry chapter 7 atomic structure and periodicity
Anatomy and physiology chapter 2
7 ionic and metallic bonding practice problems
Quantum and nuclear physics
Pron and cons
Conclusion on topic family