Community ecology and ecosystem ecology student directed notes
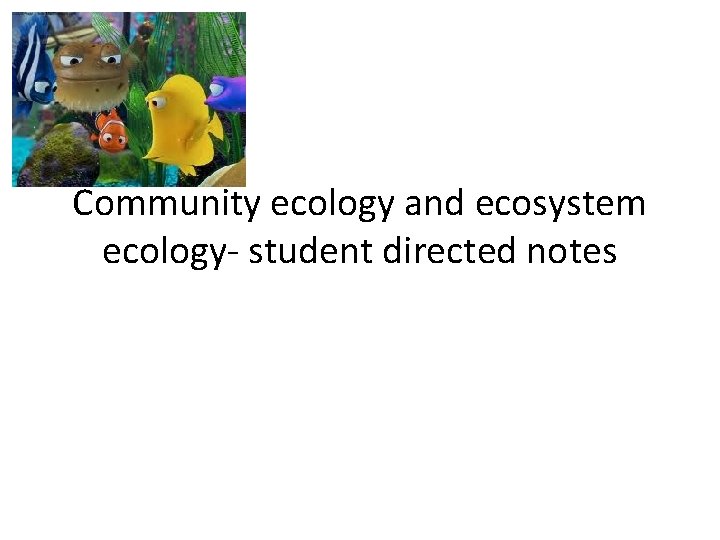
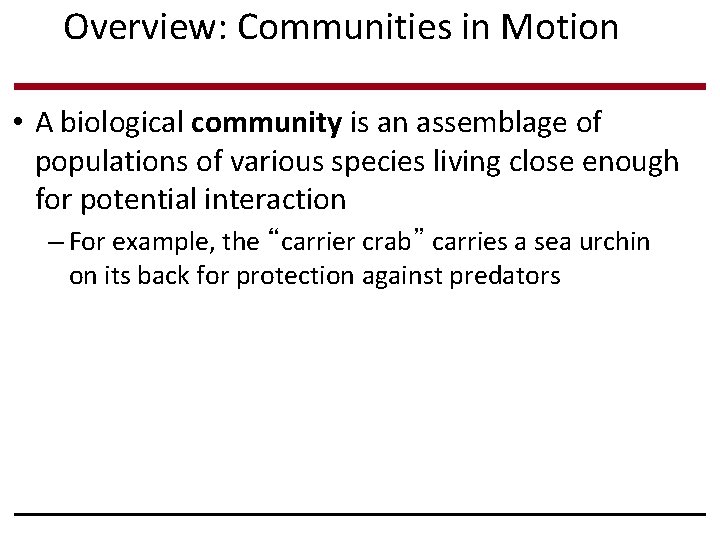
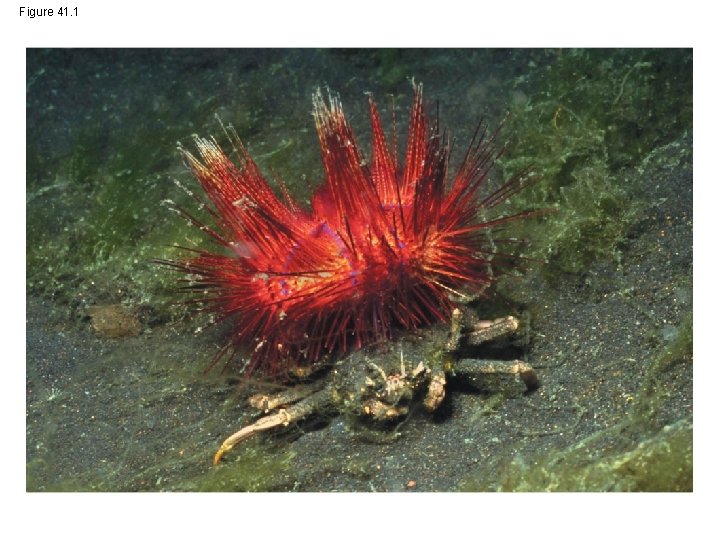
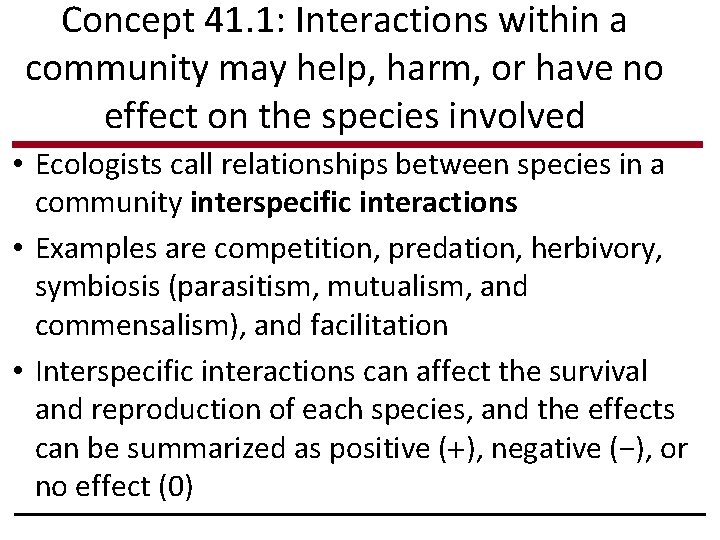
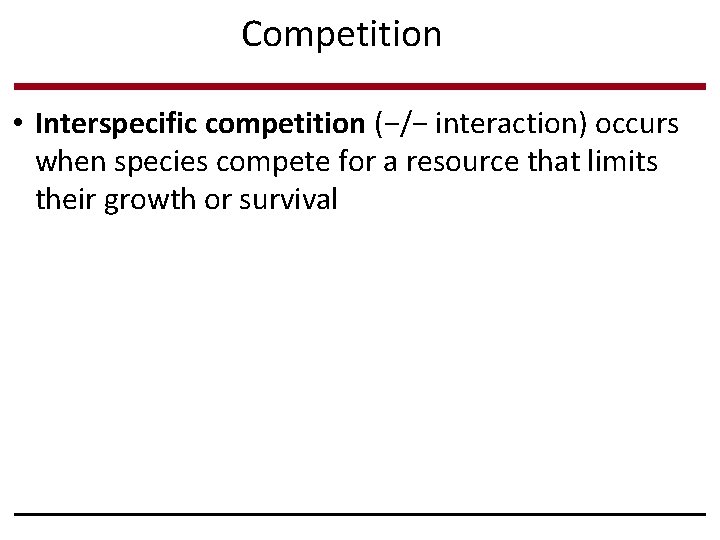
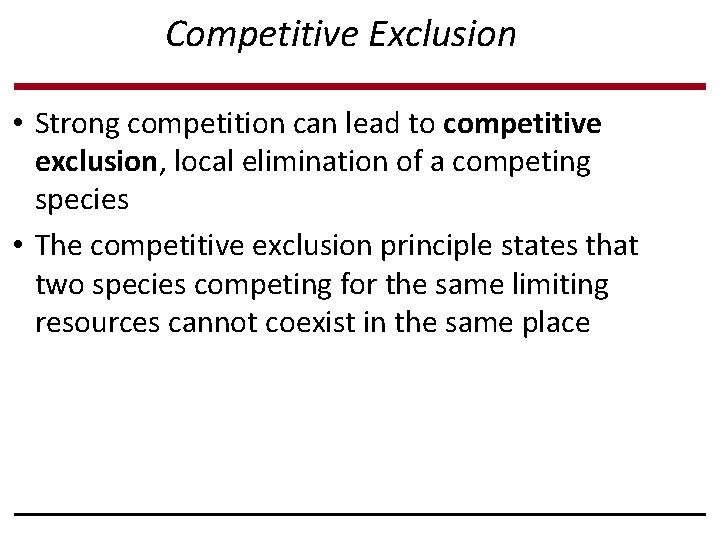
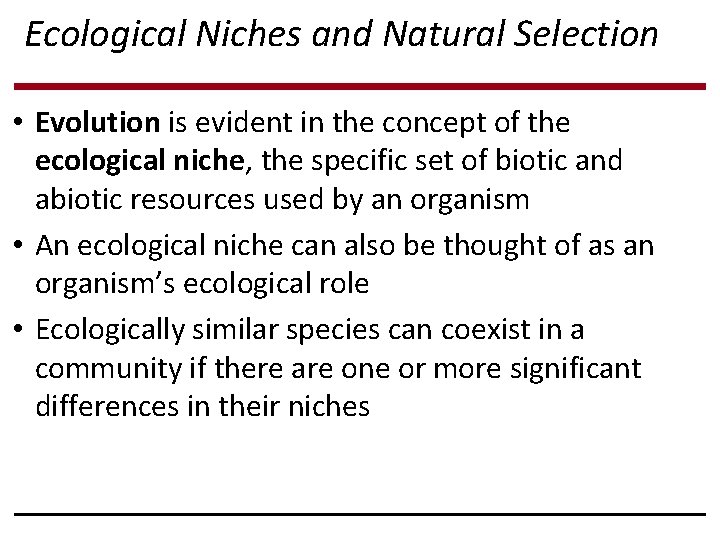
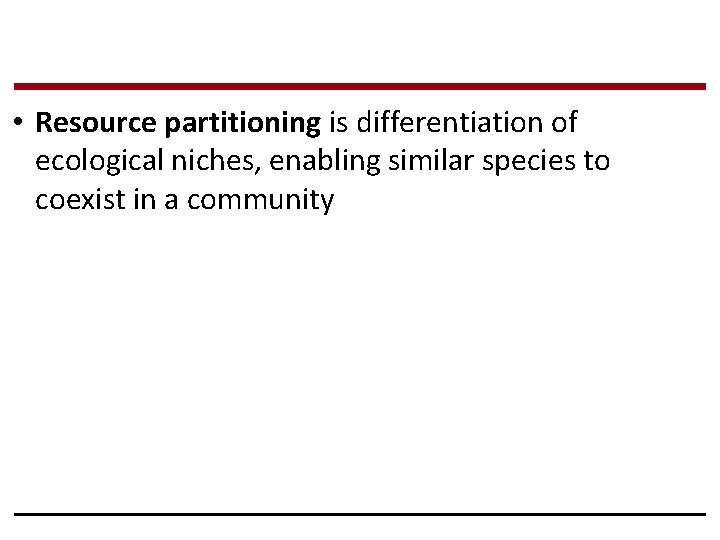
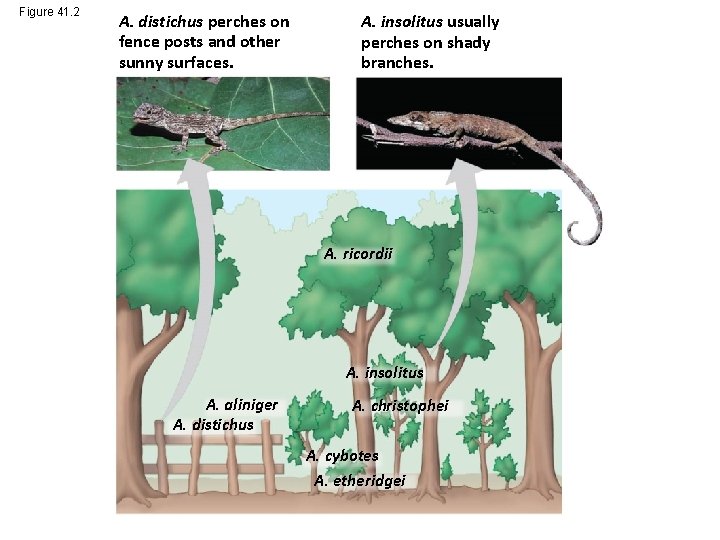
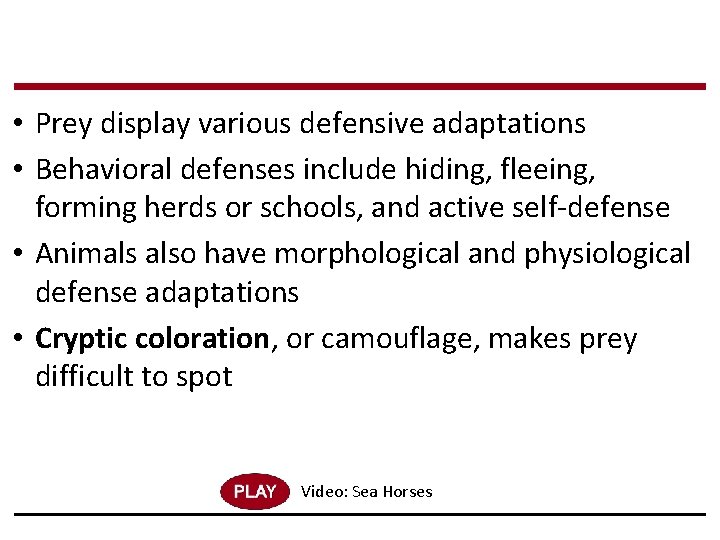
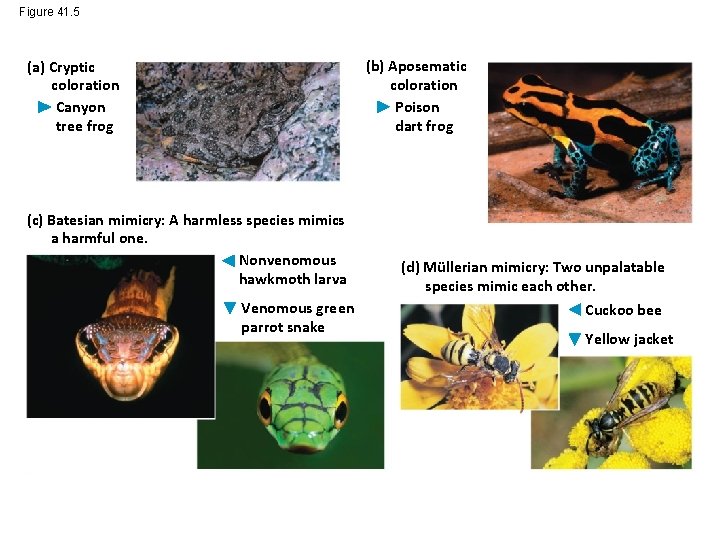
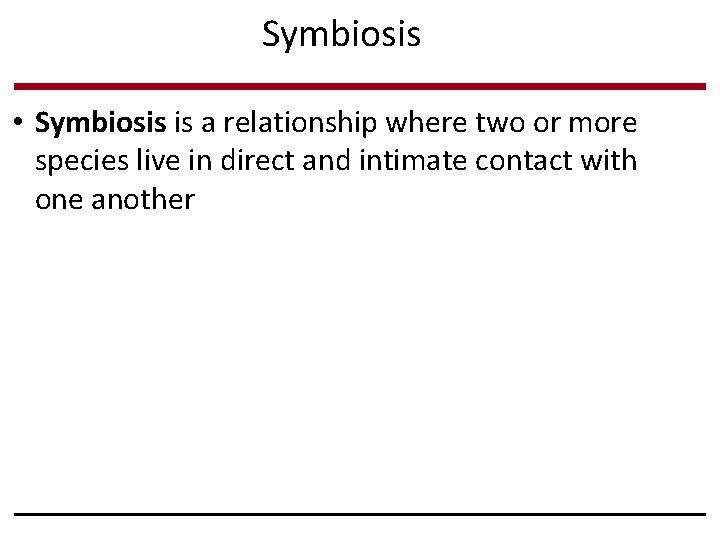
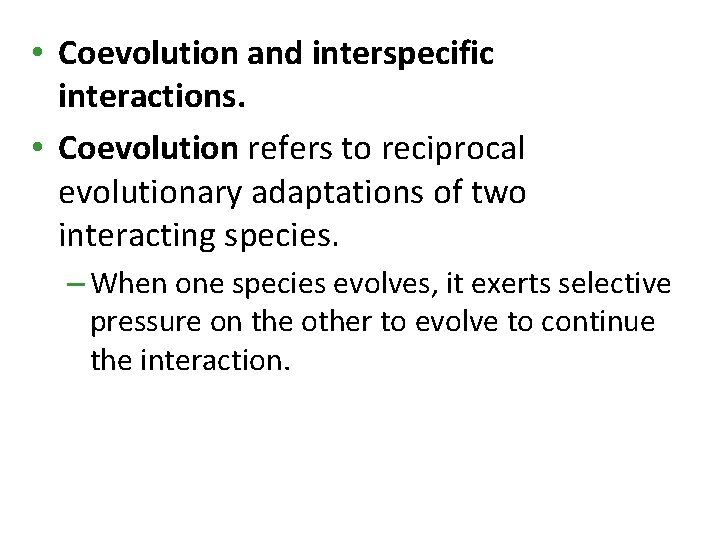
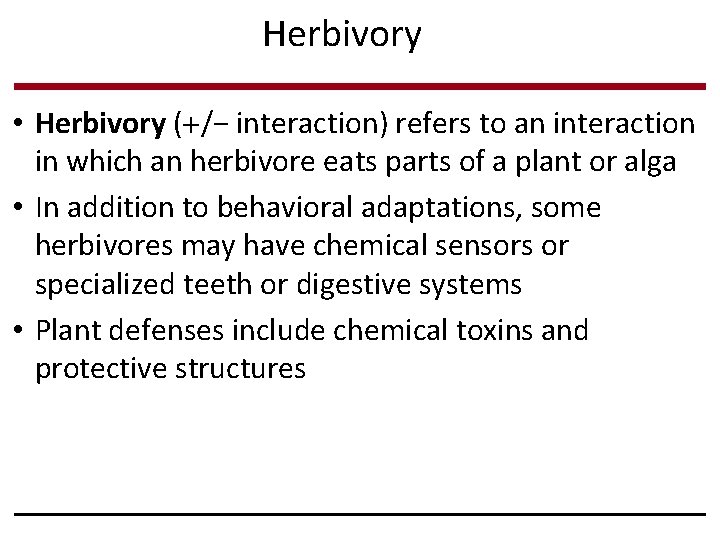
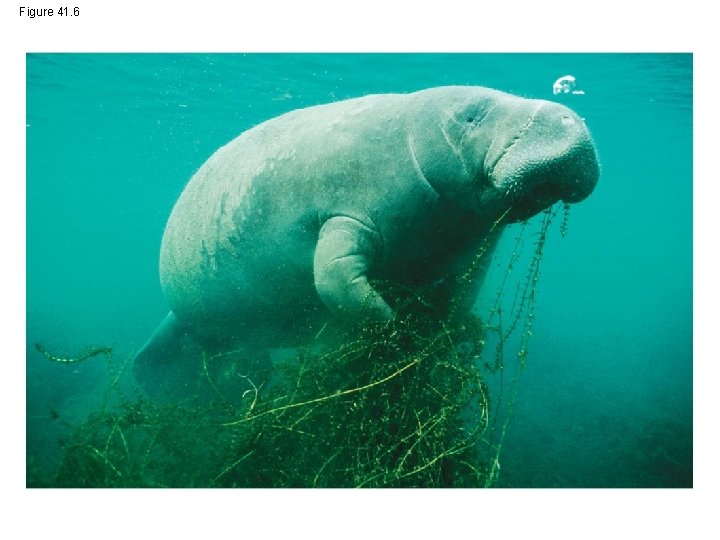
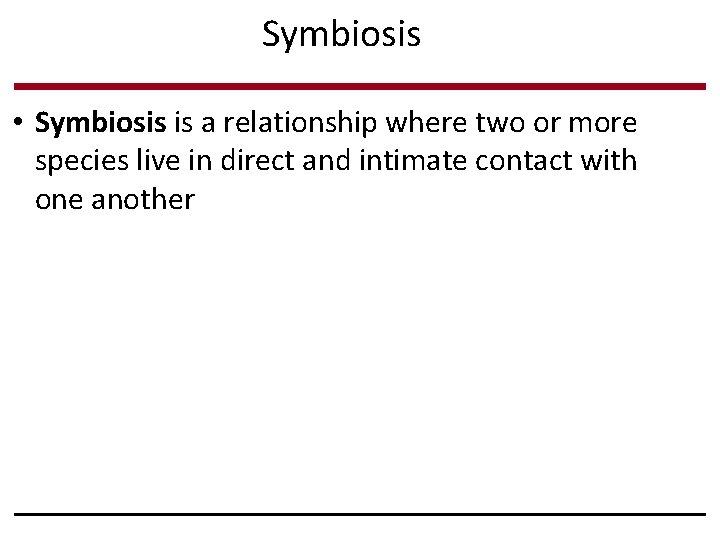
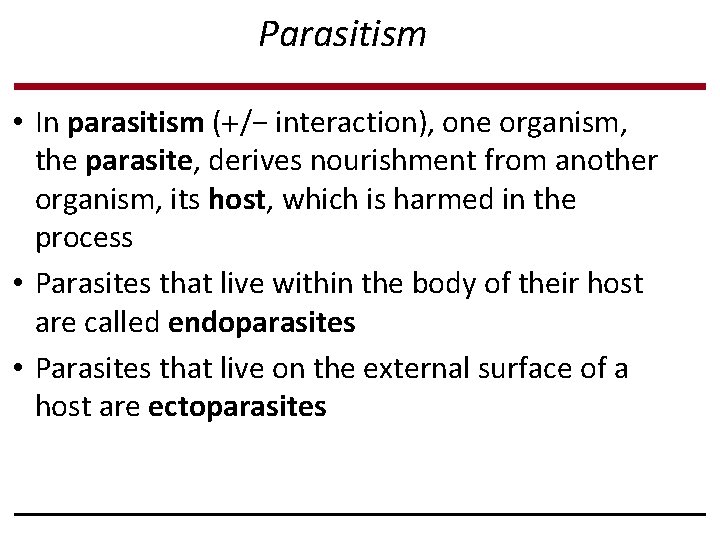
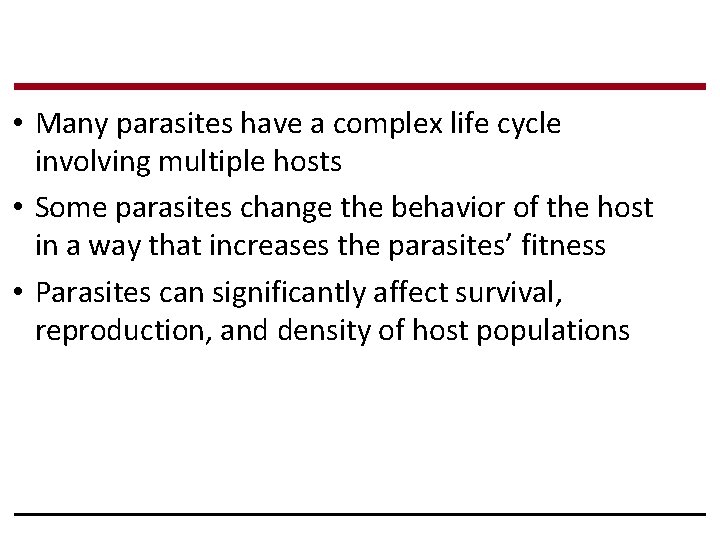
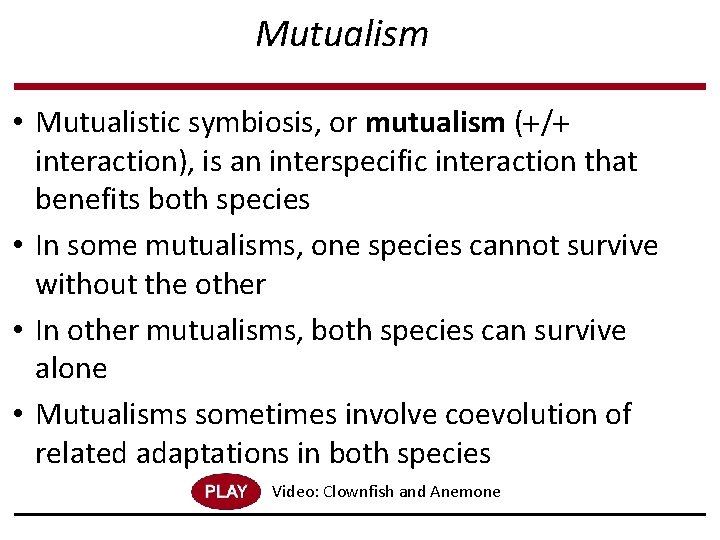
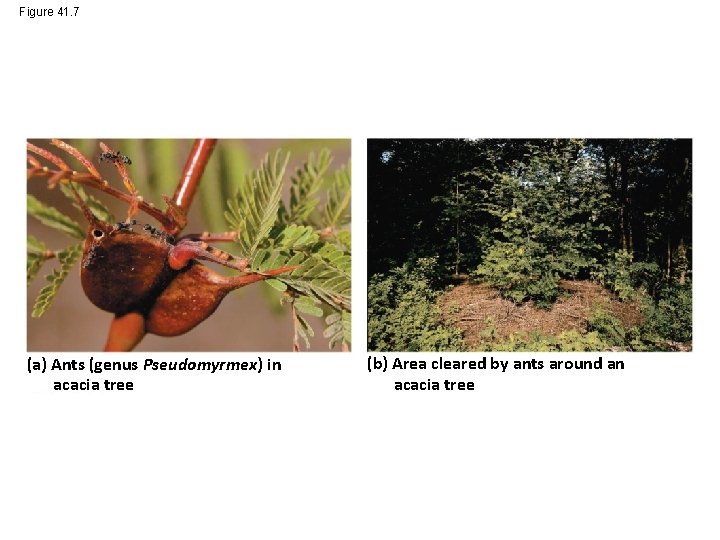
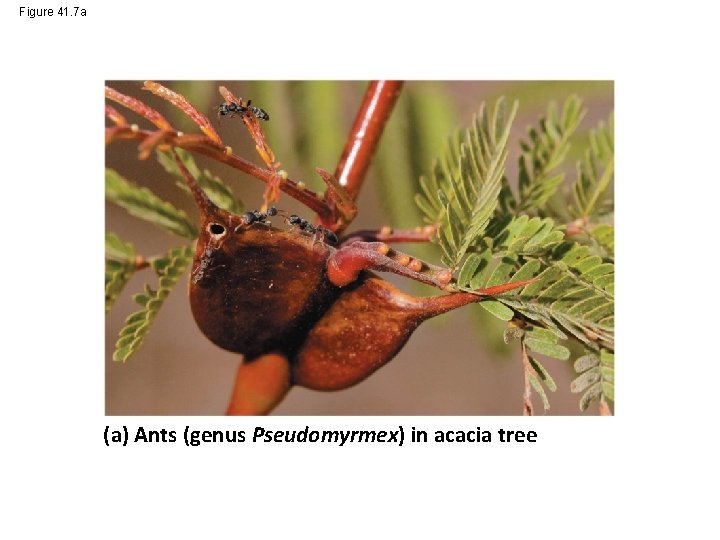
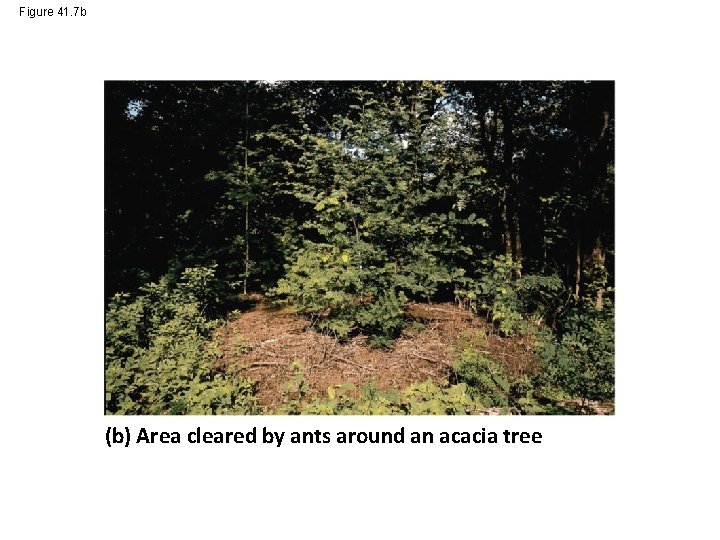
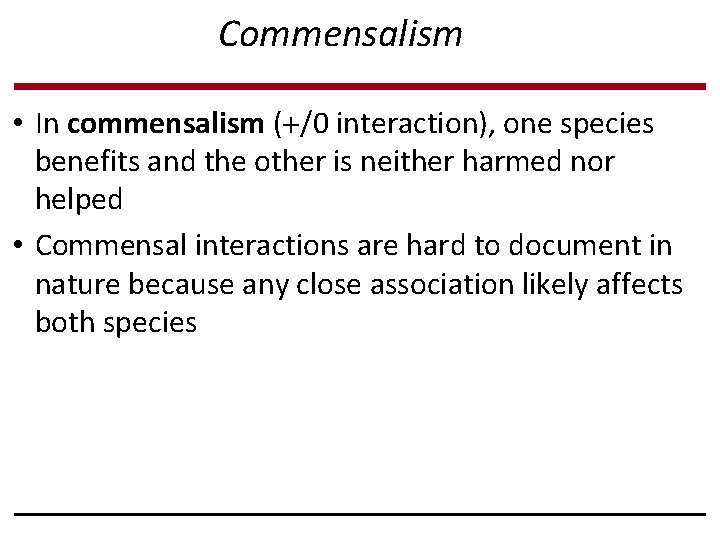
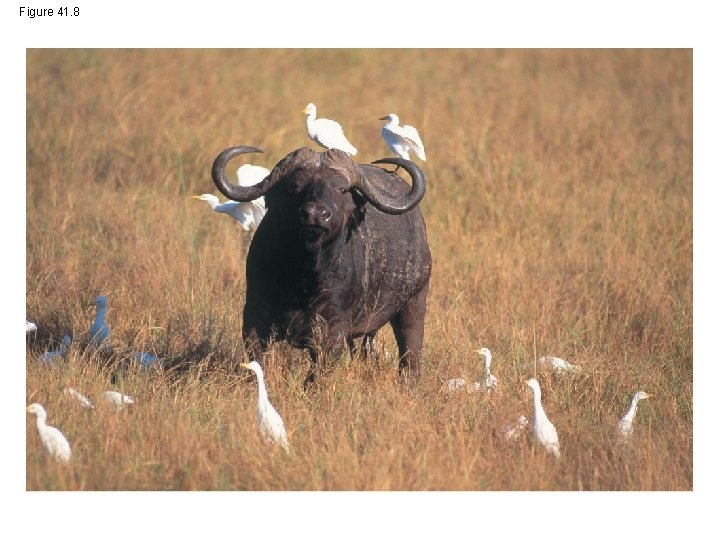
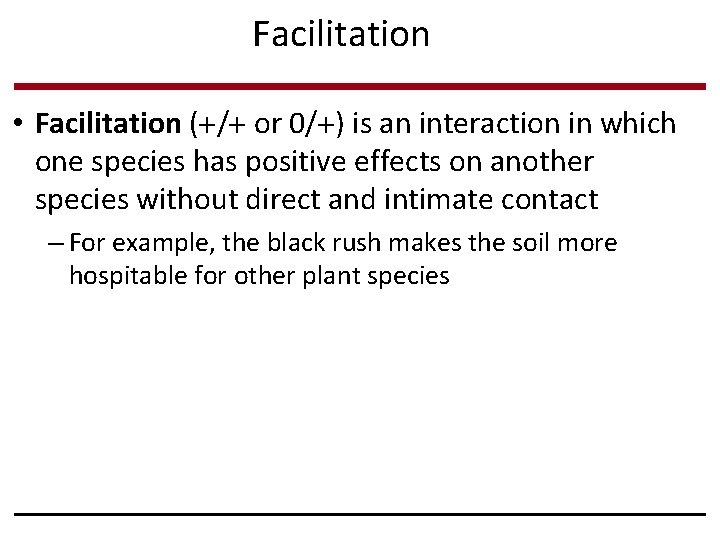
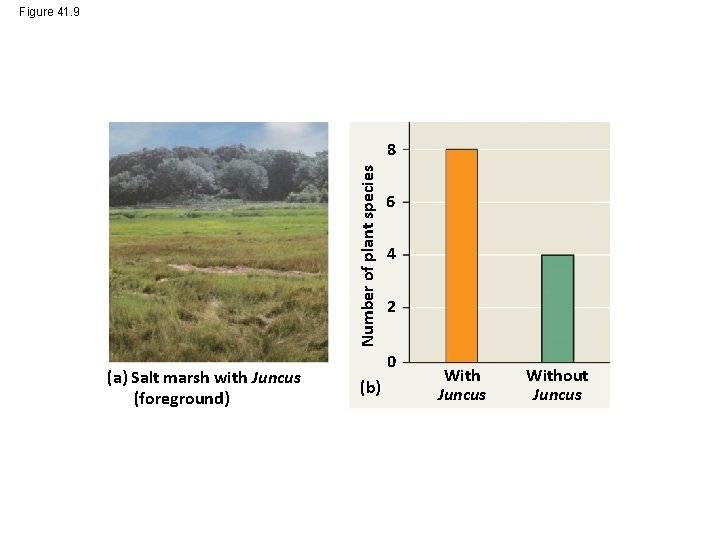
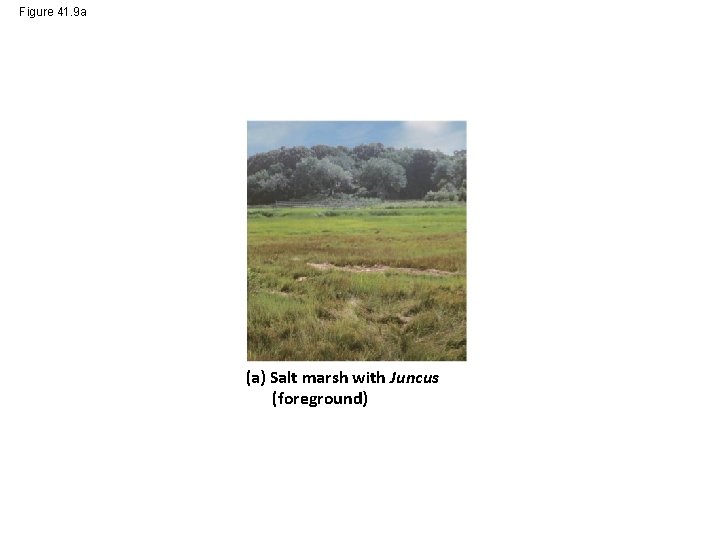
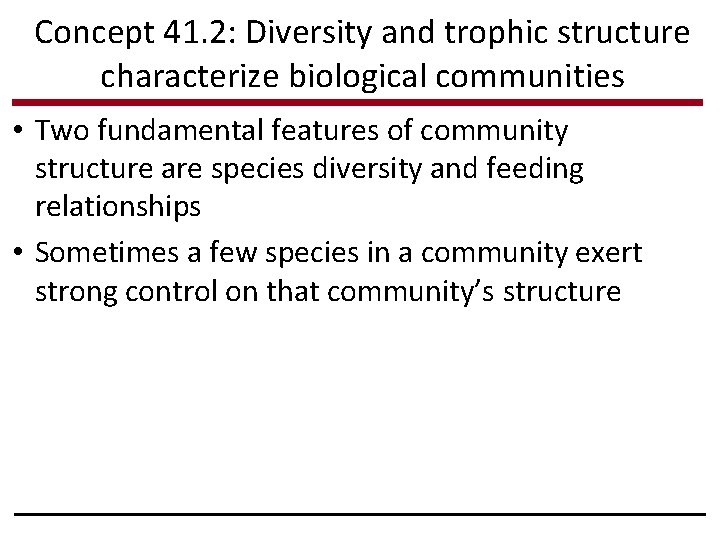
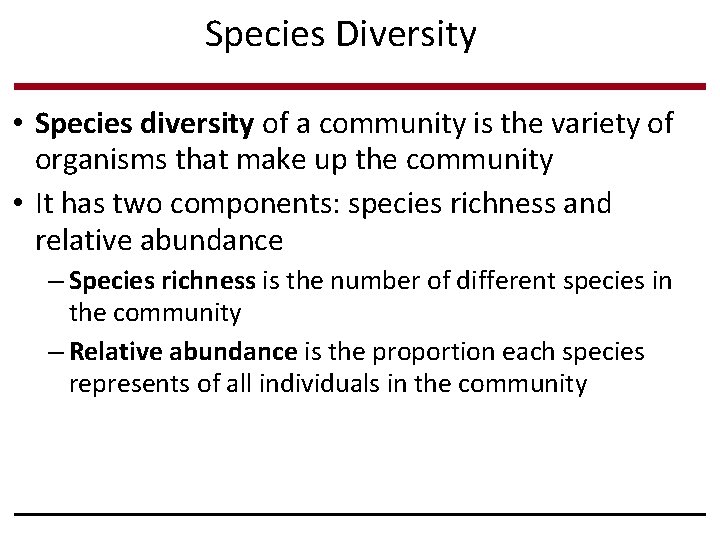
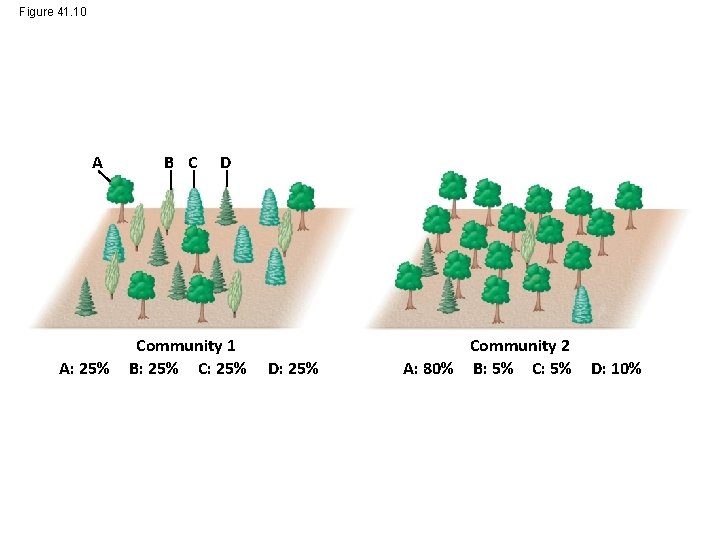
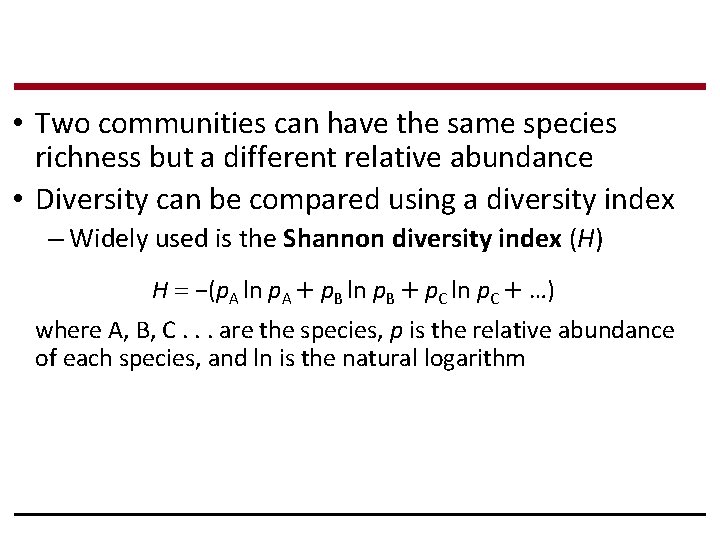
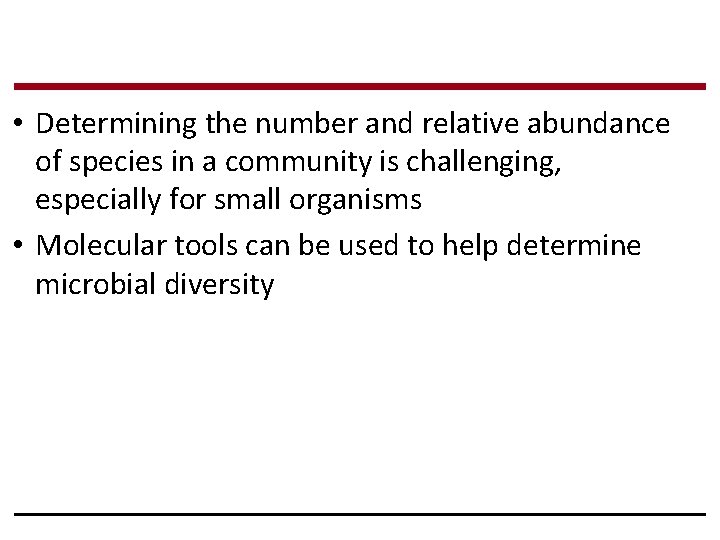
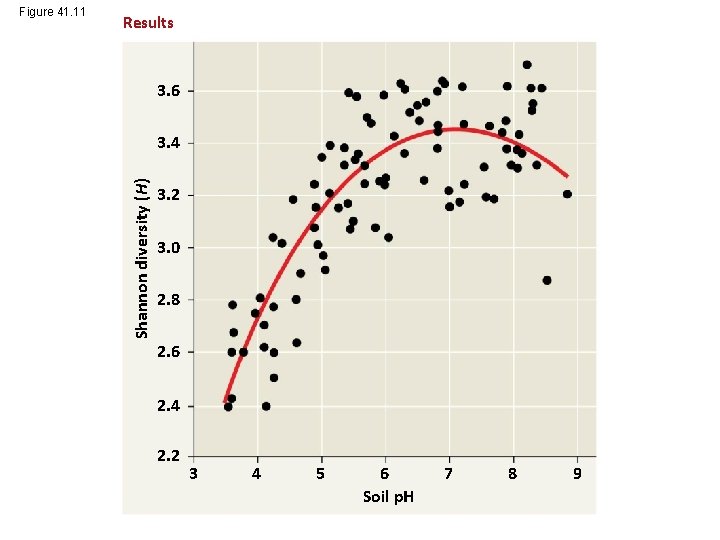
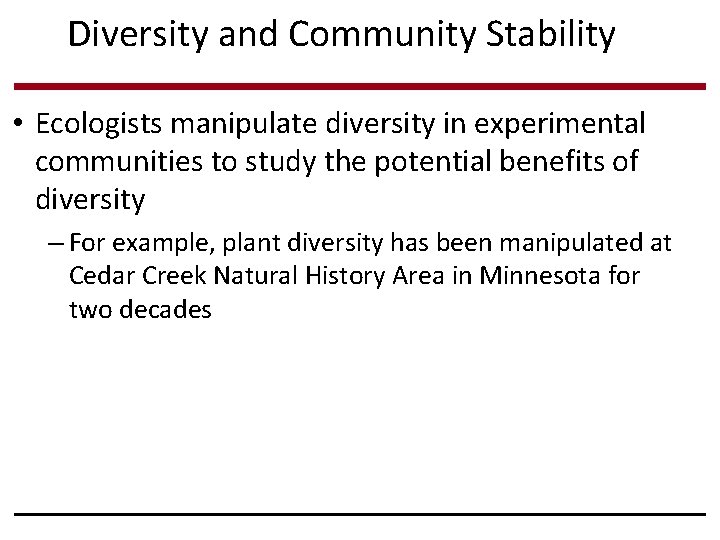
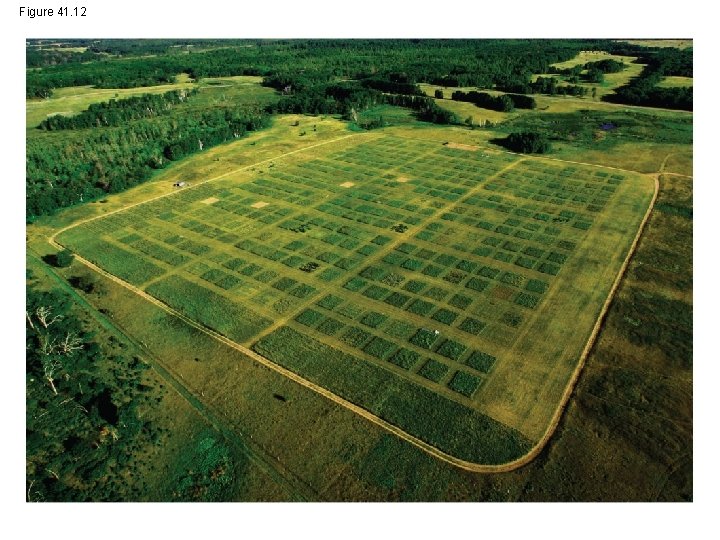
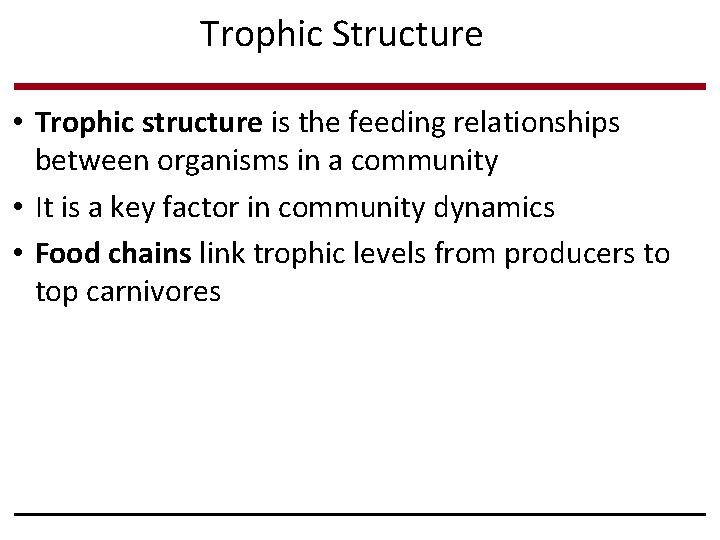
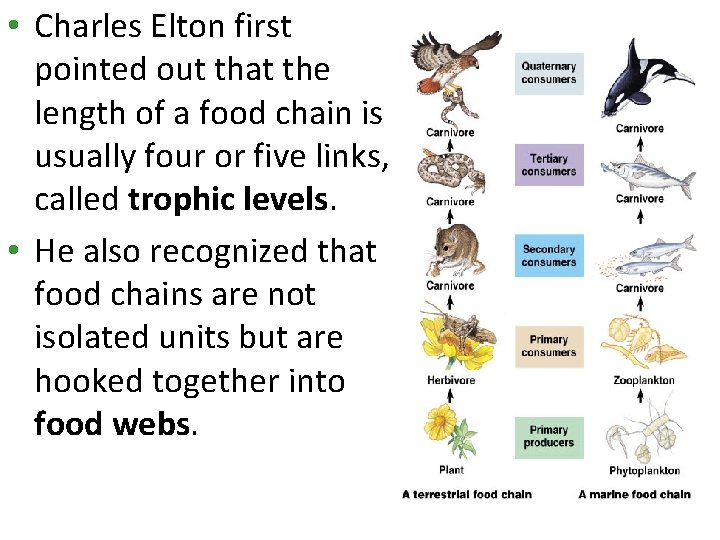
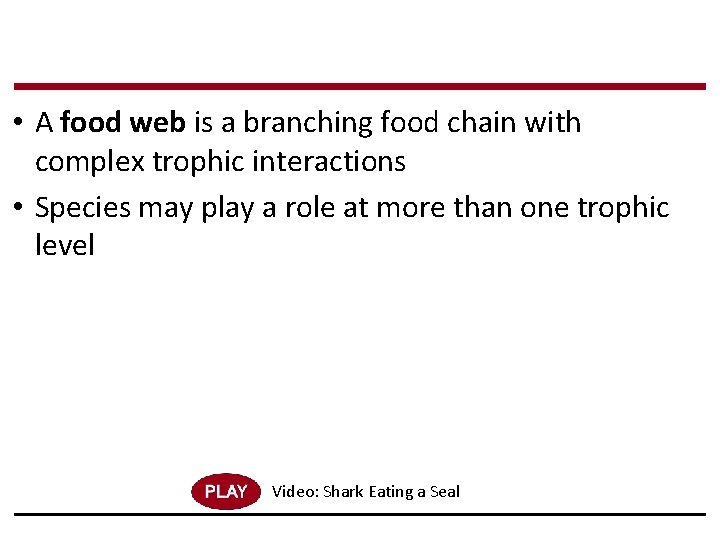
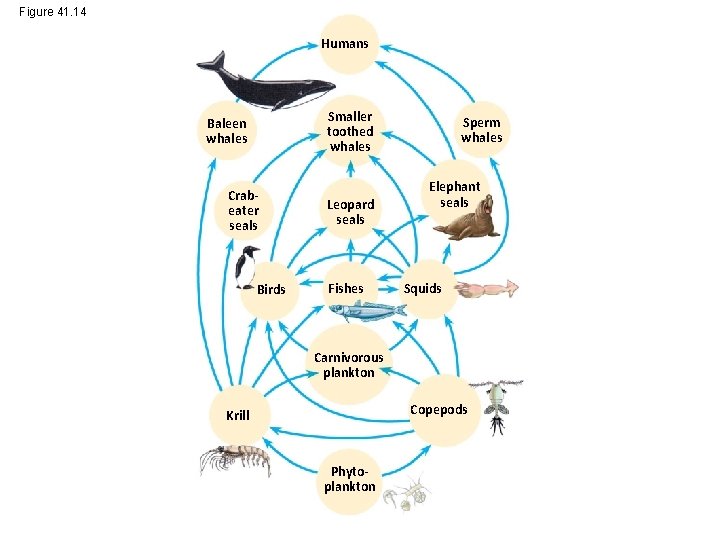
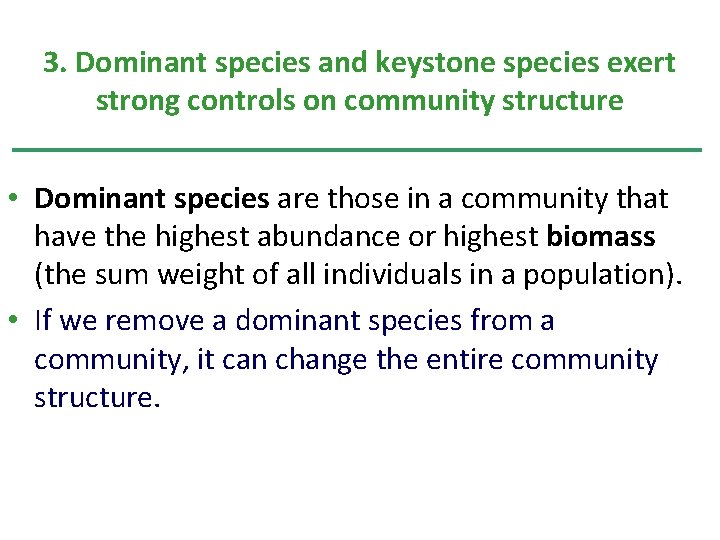
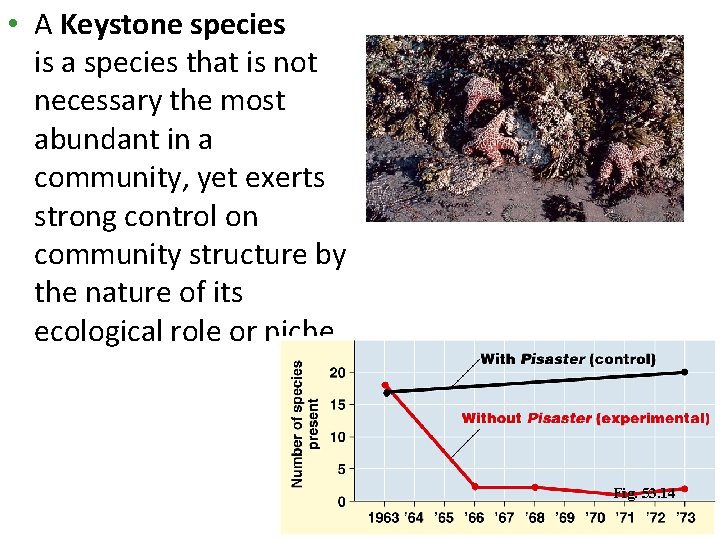
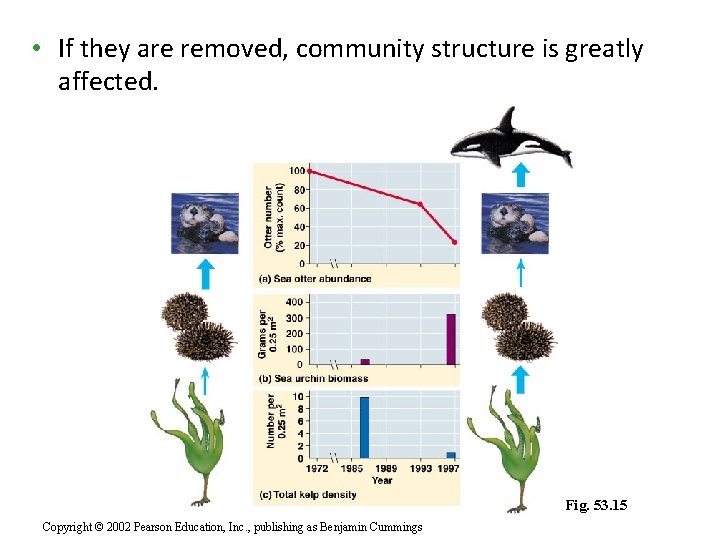
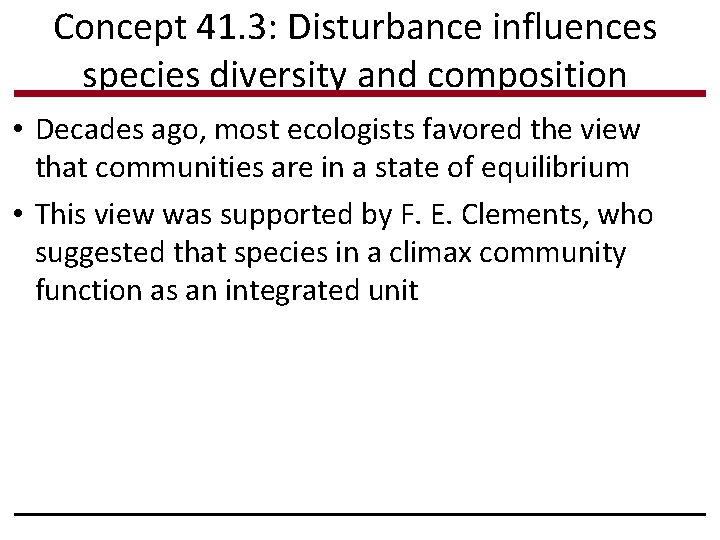
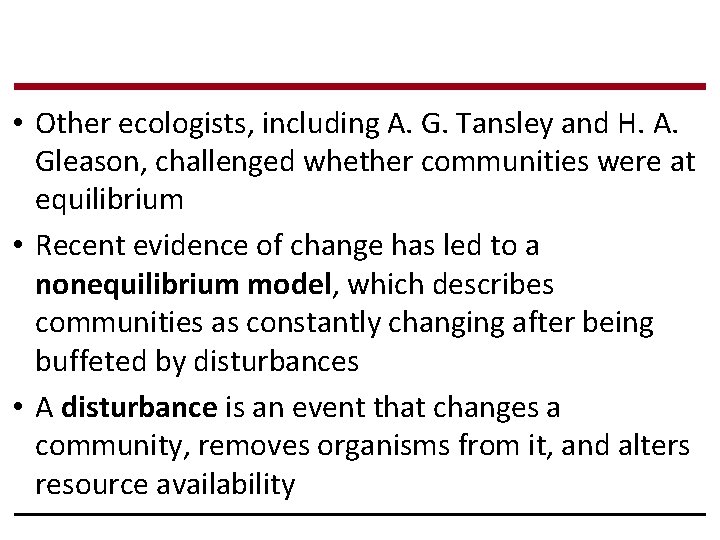
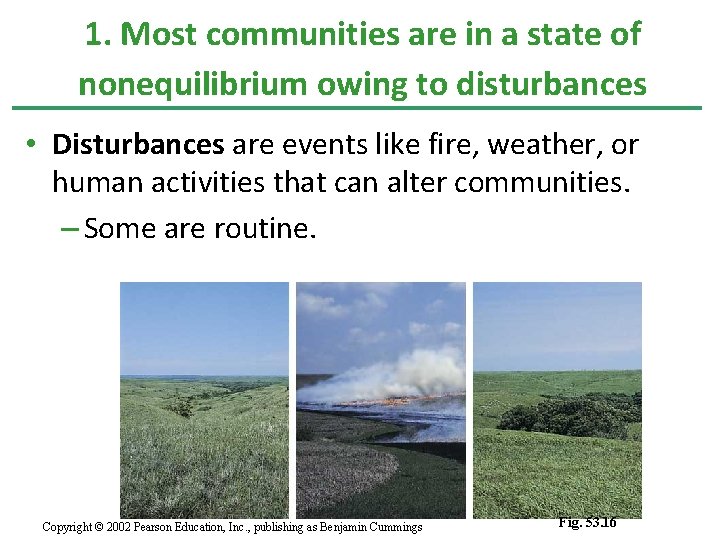
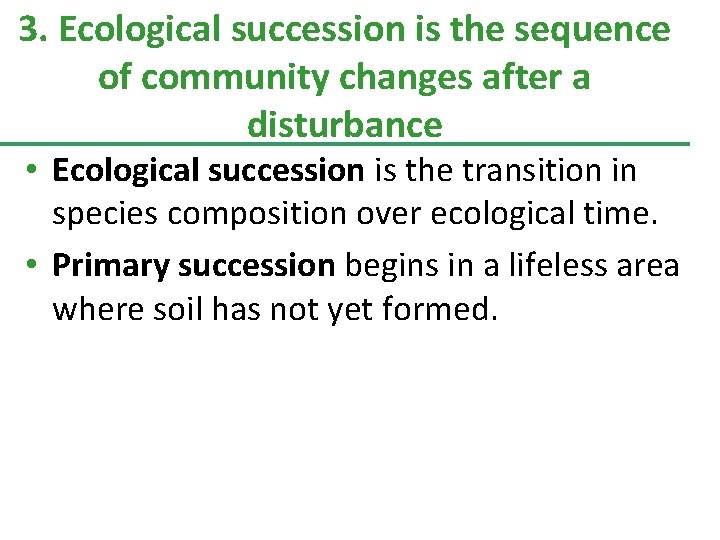
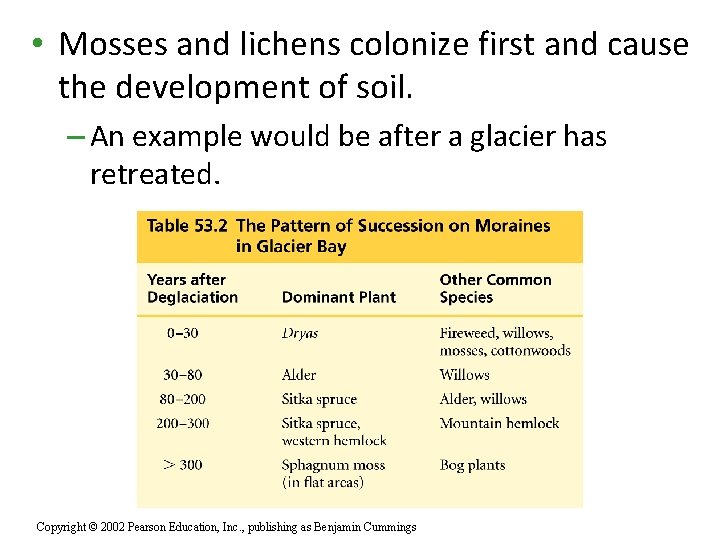
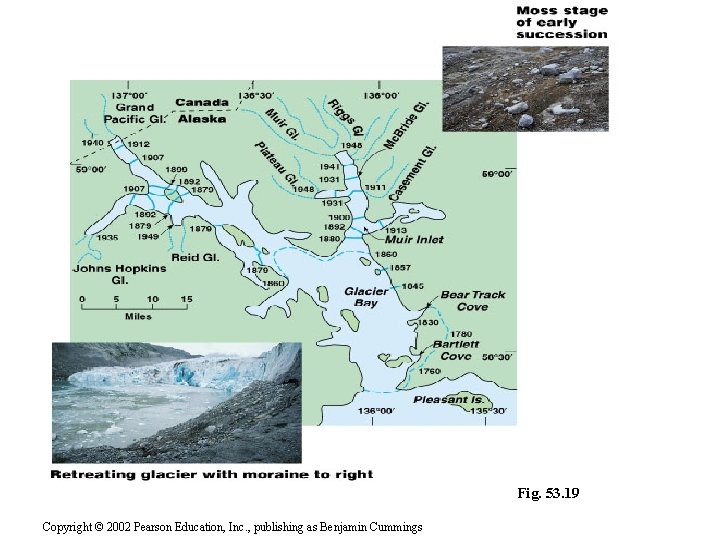
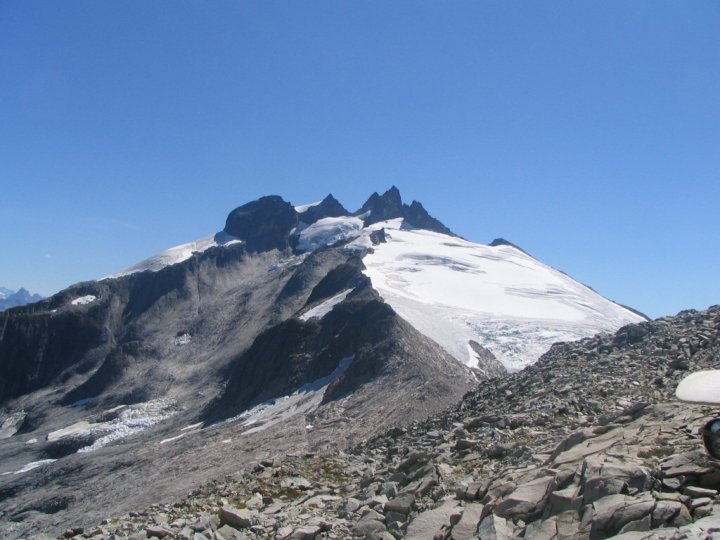
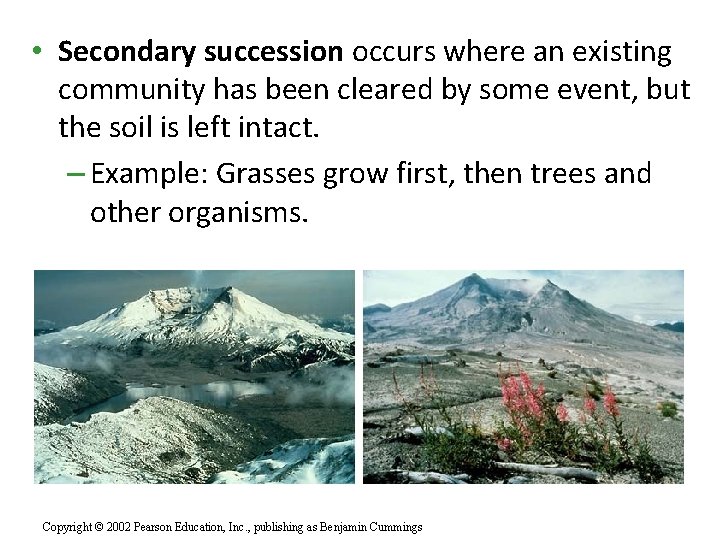
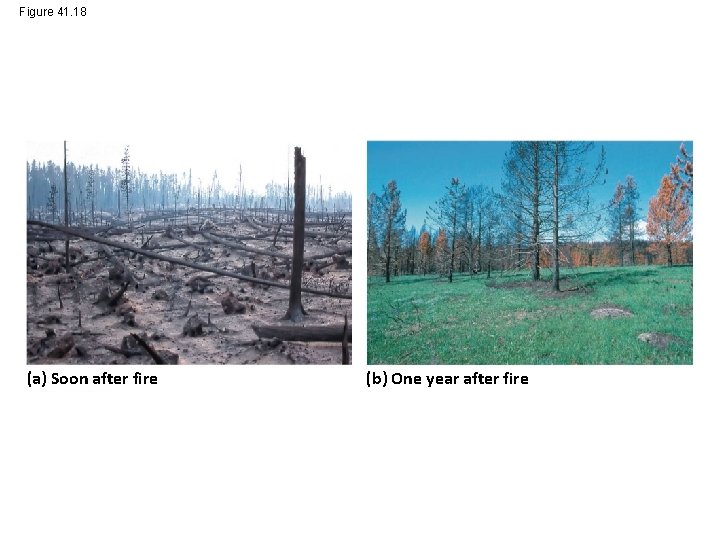
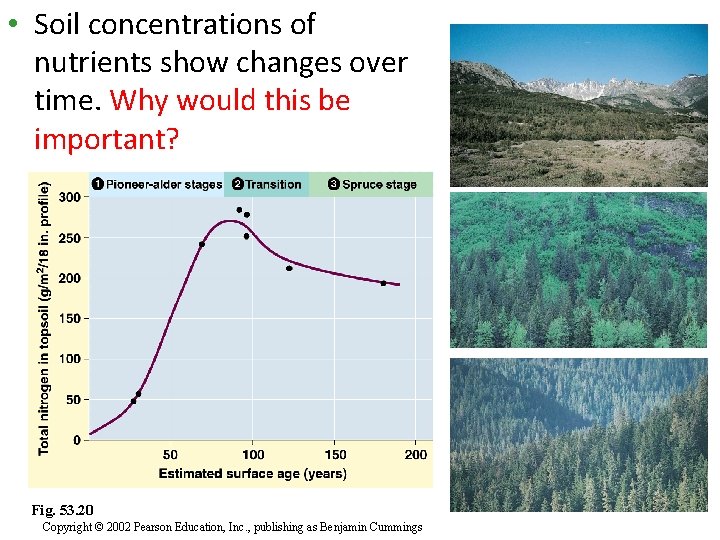
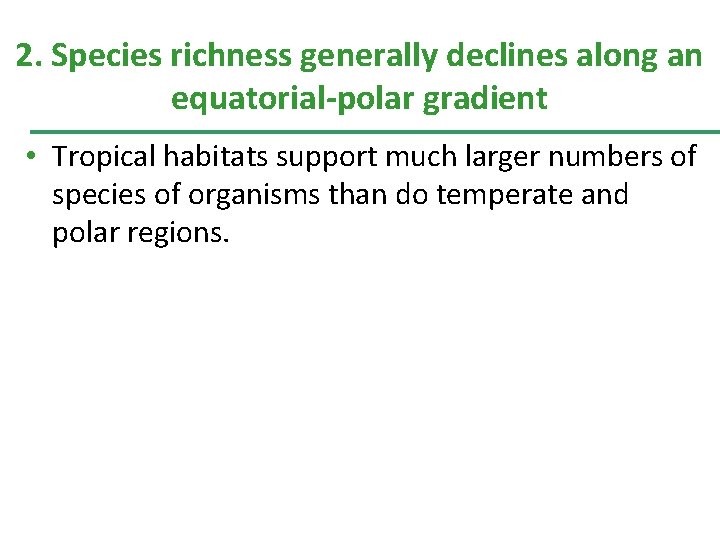
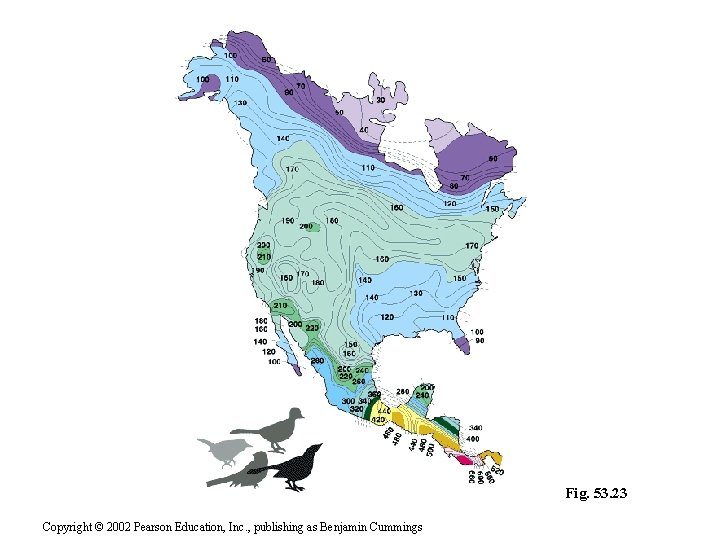
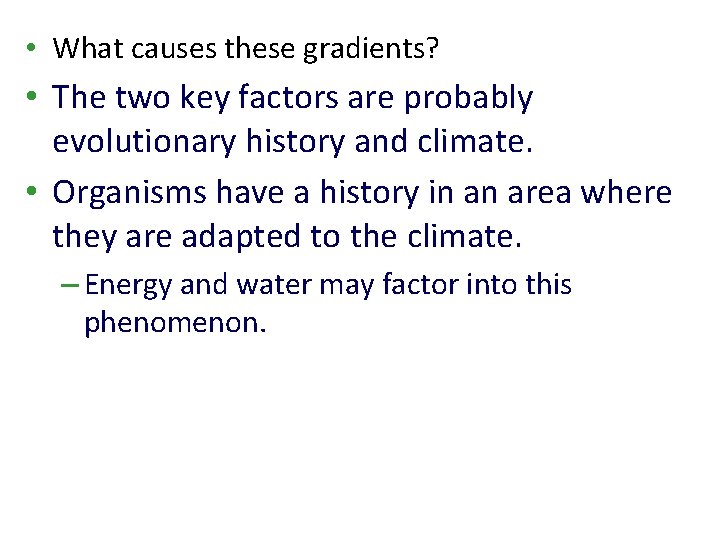
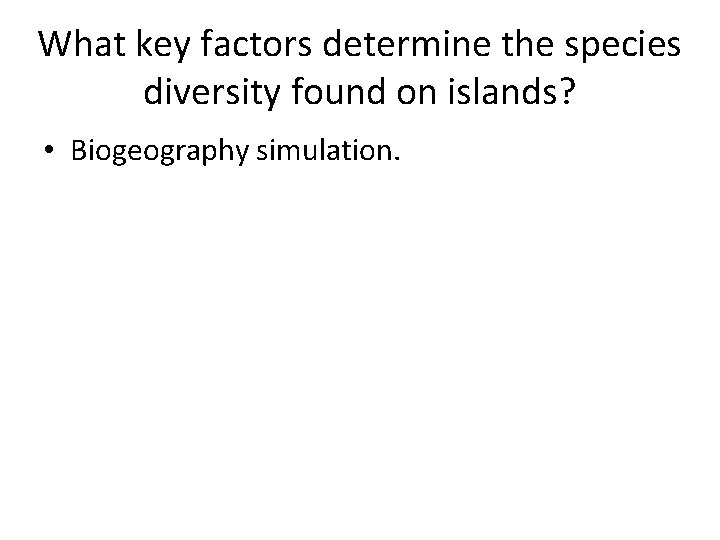
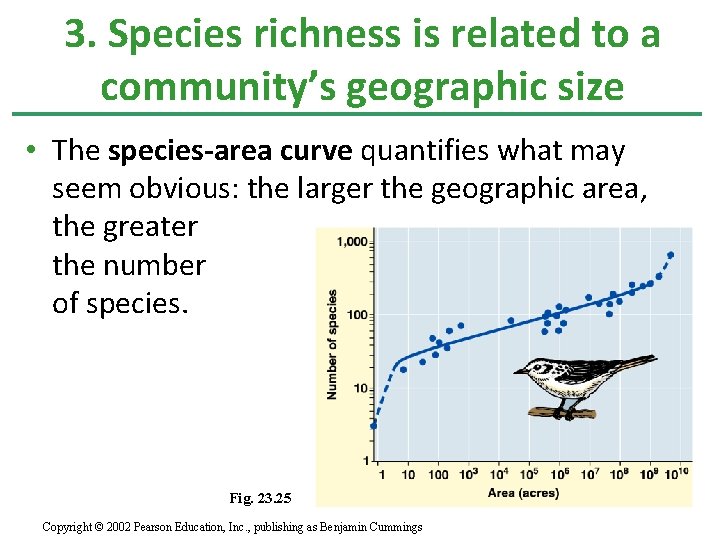
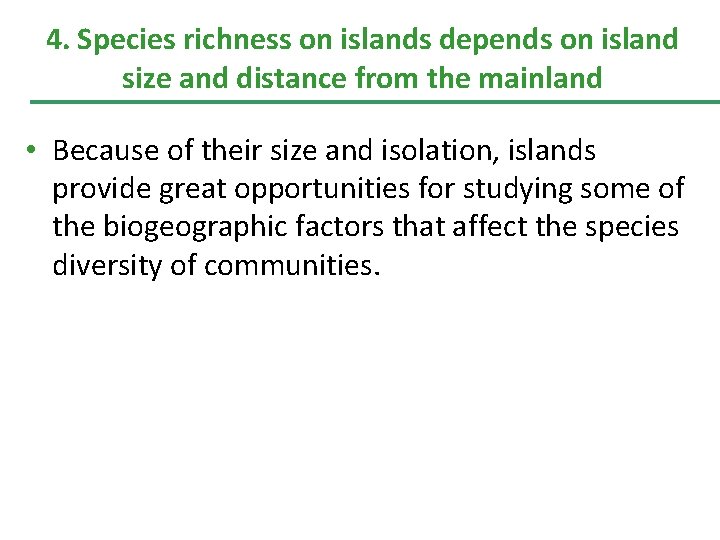
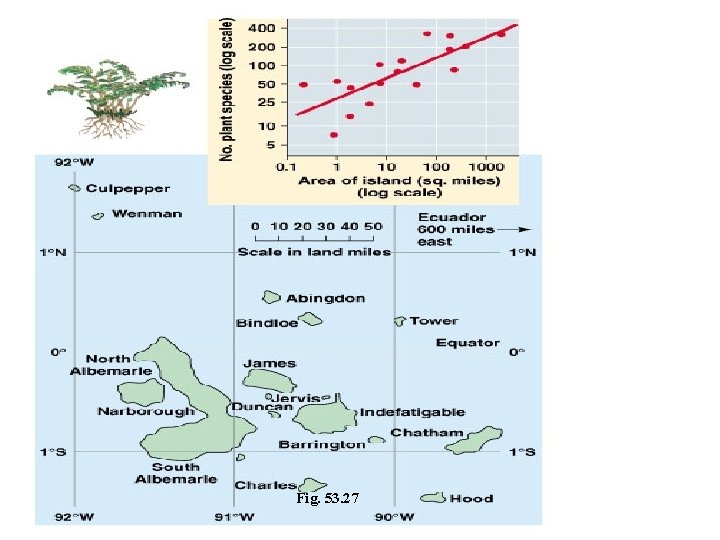
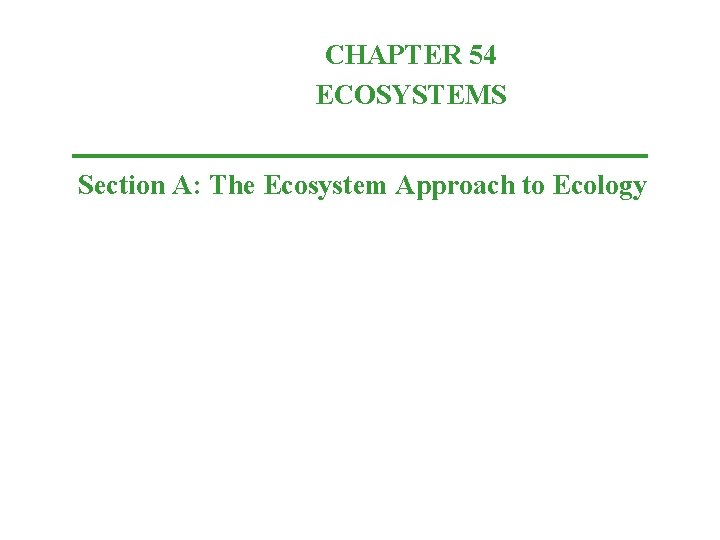
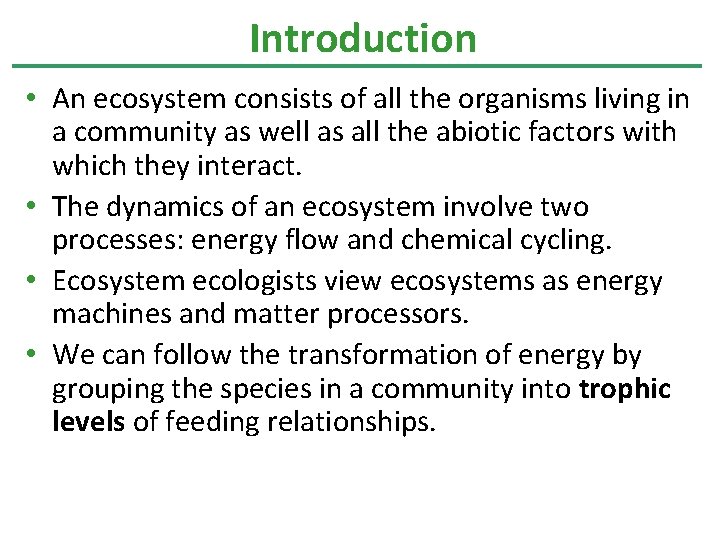
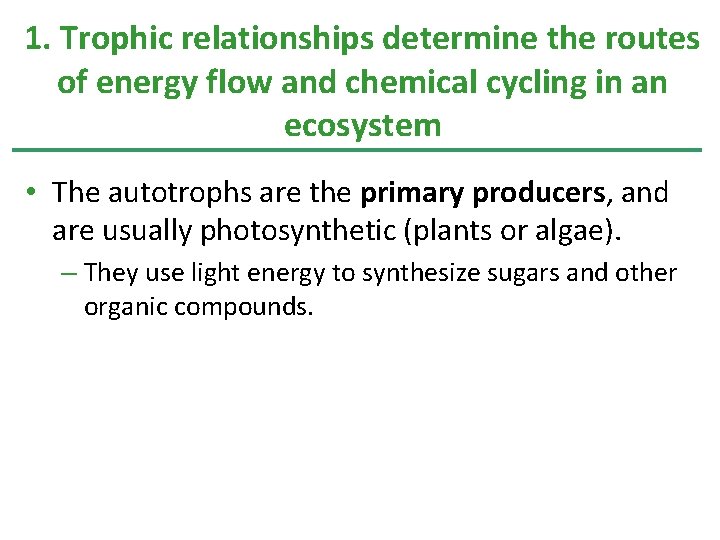
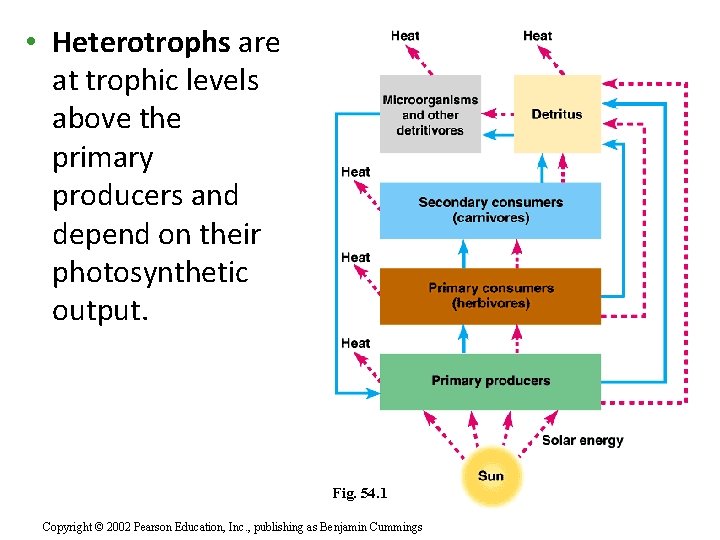
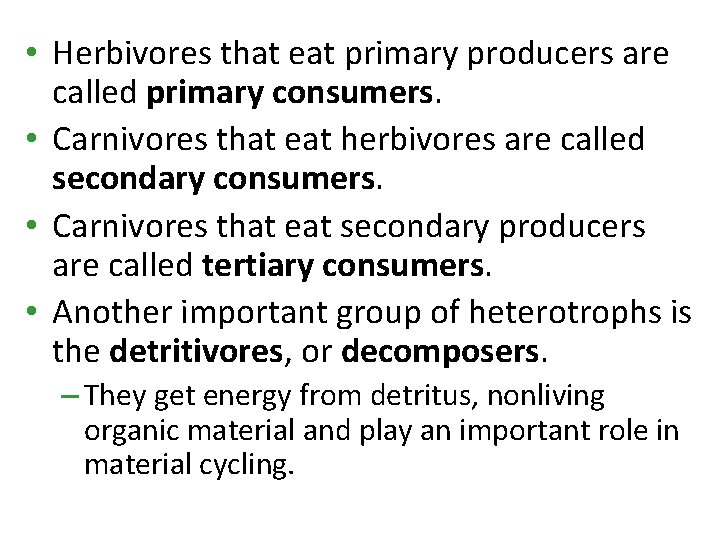
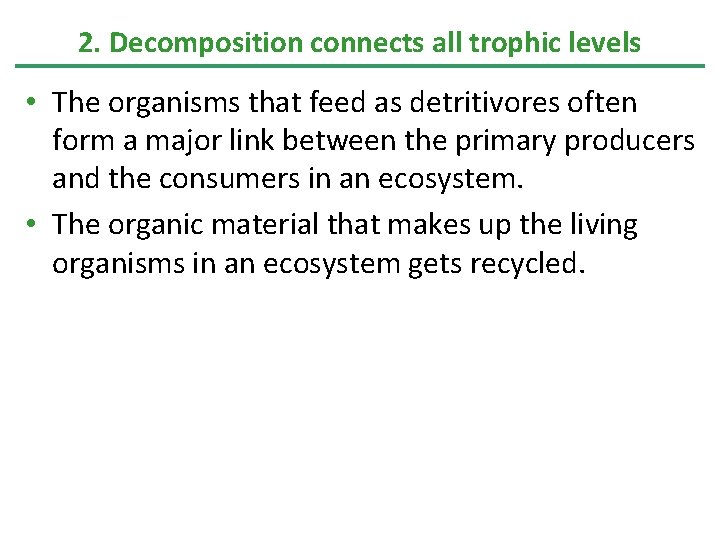
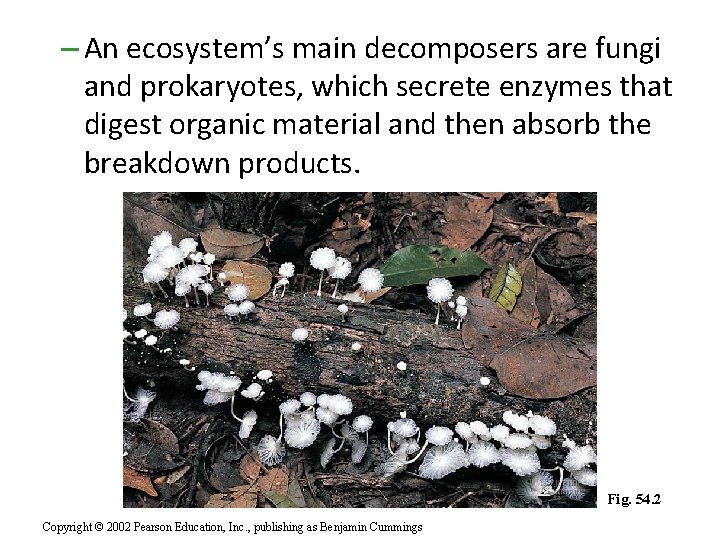
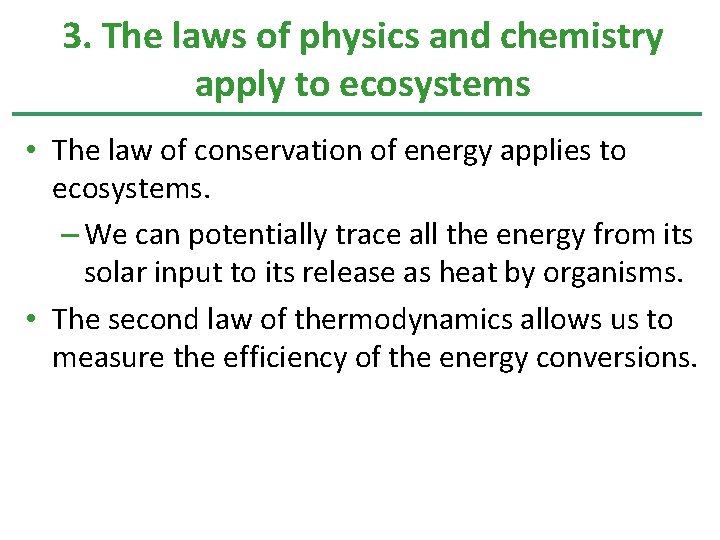
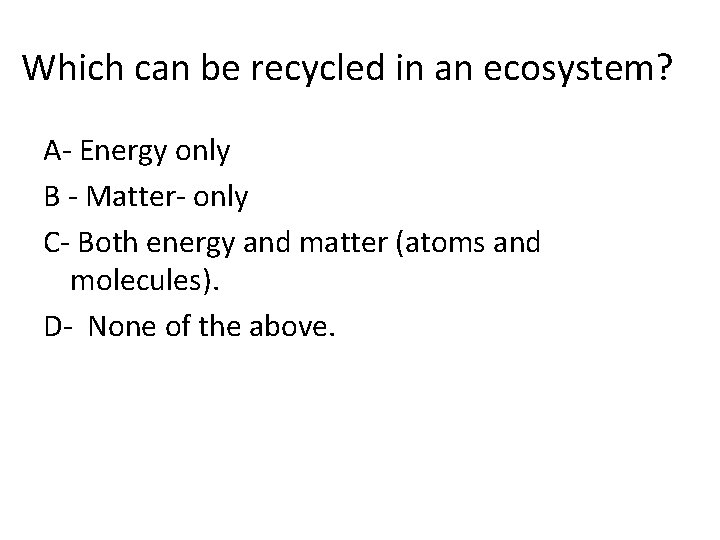
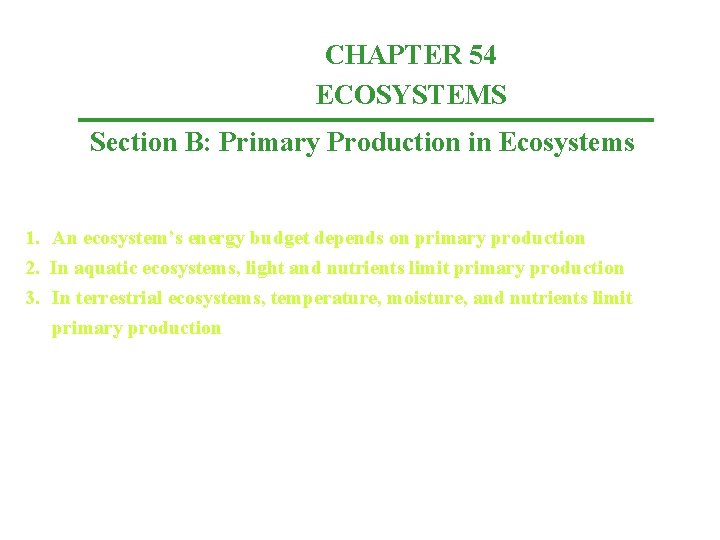
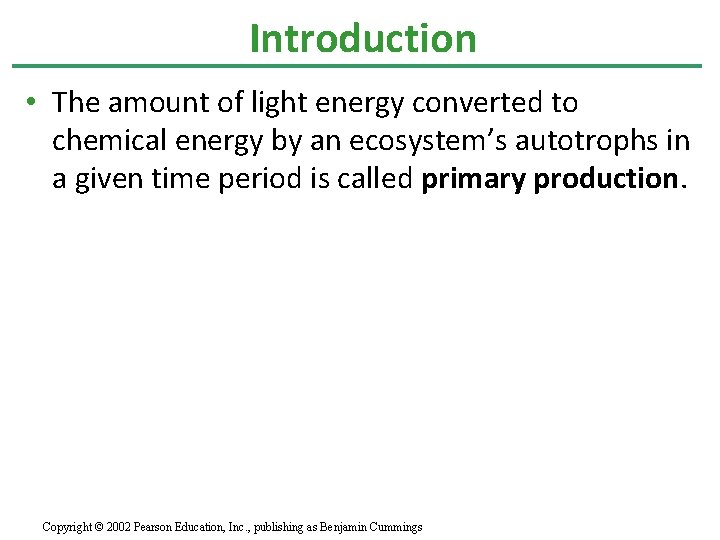
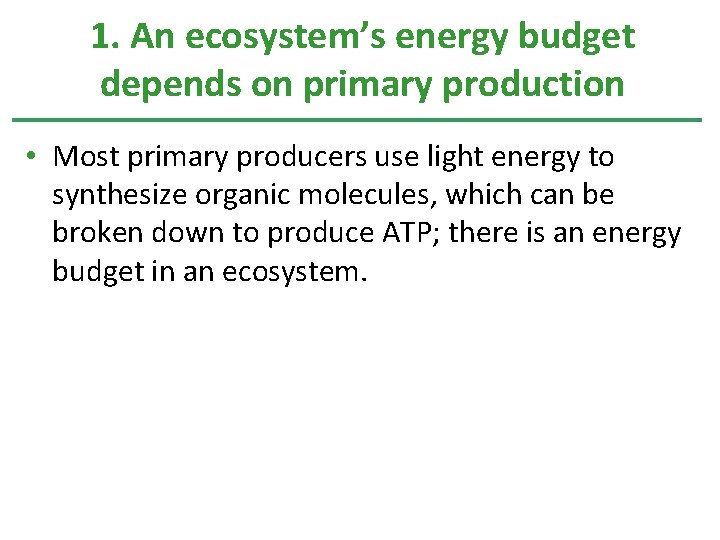
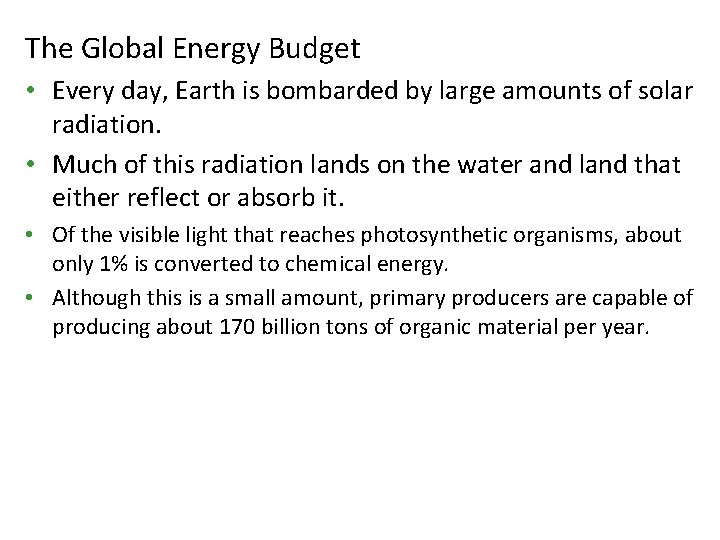
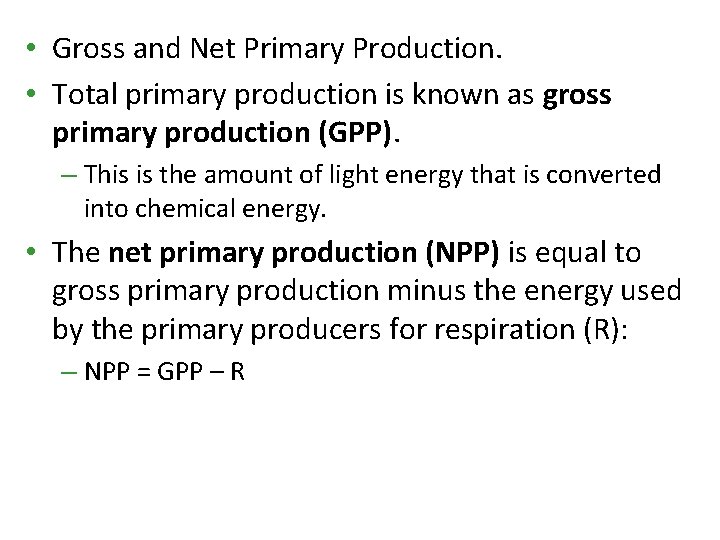
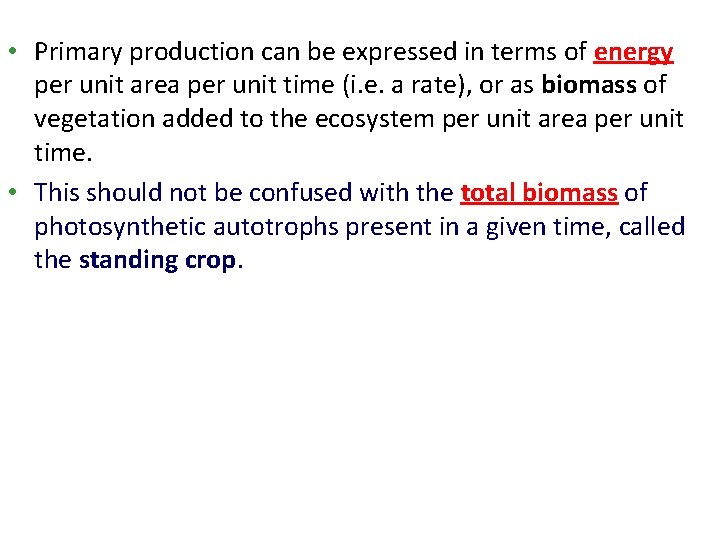
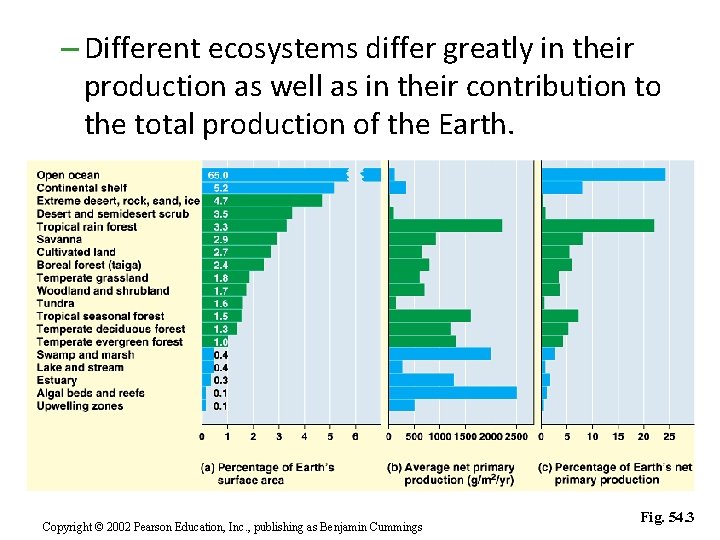
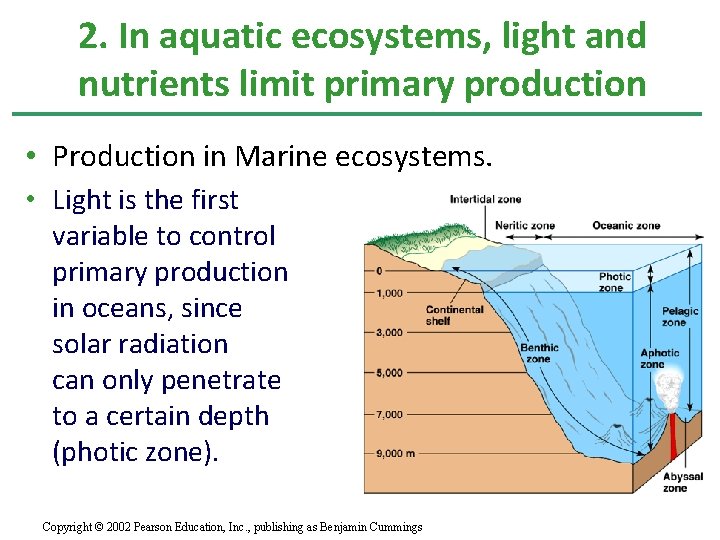
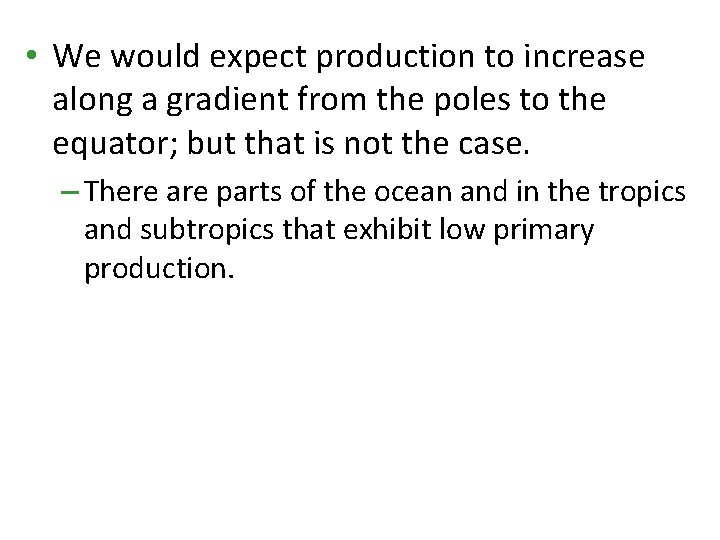
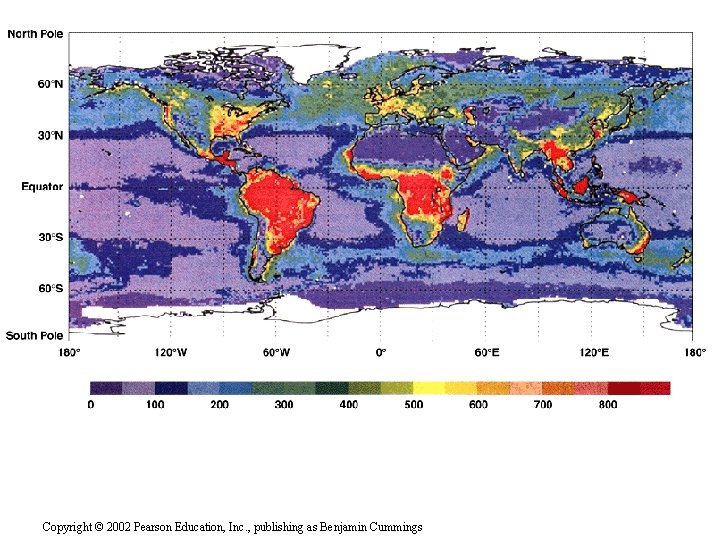
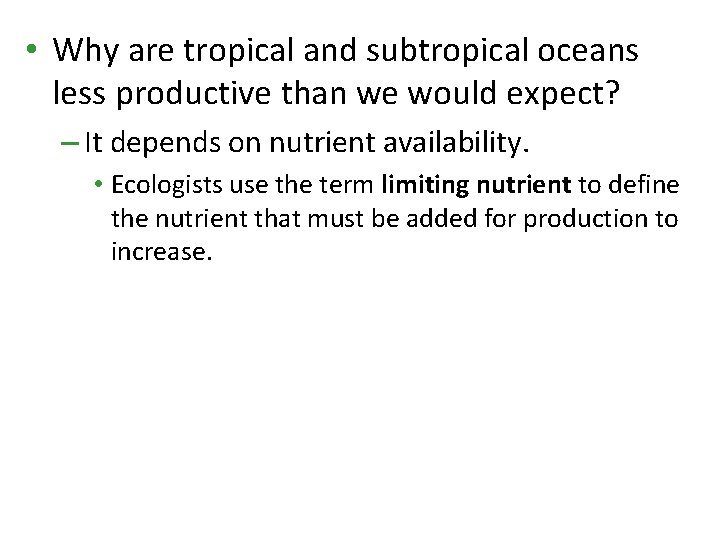
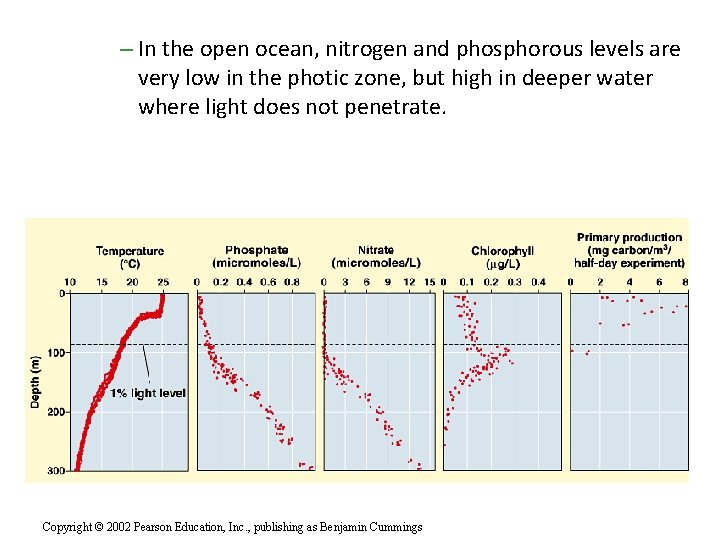
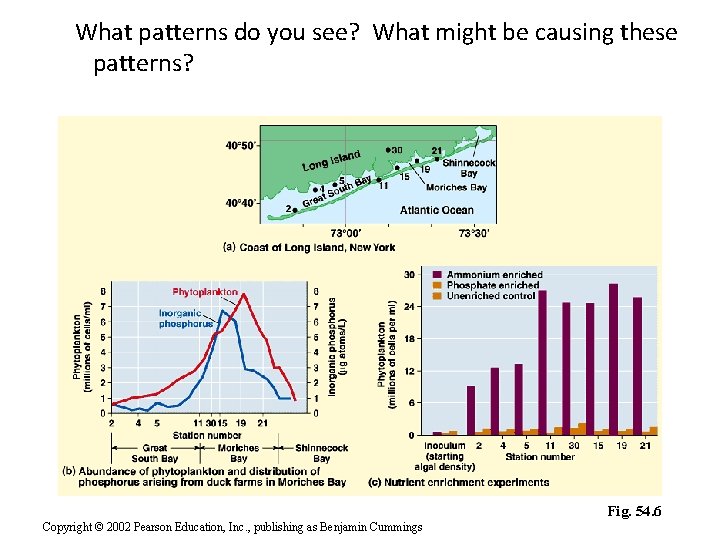
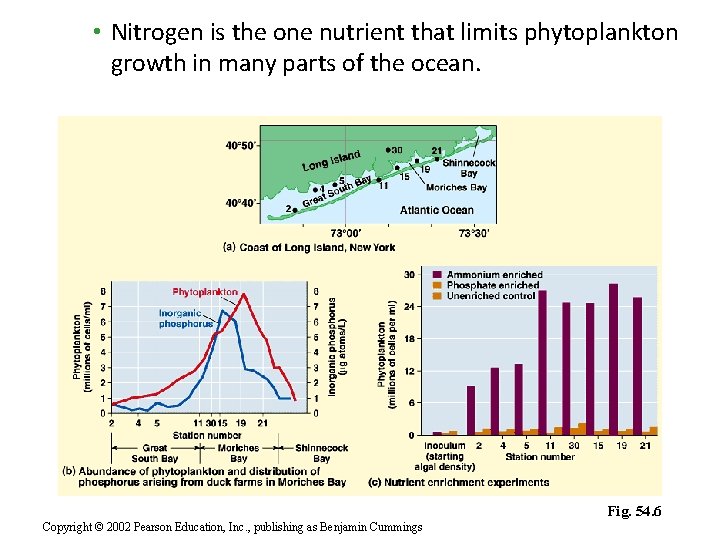
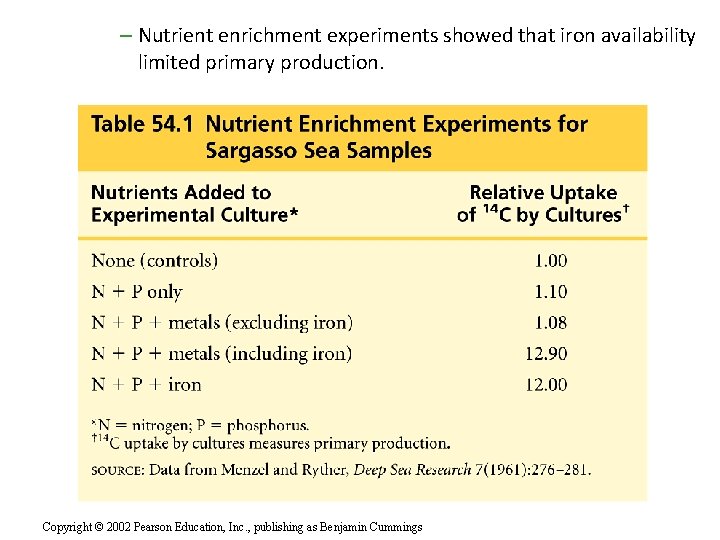
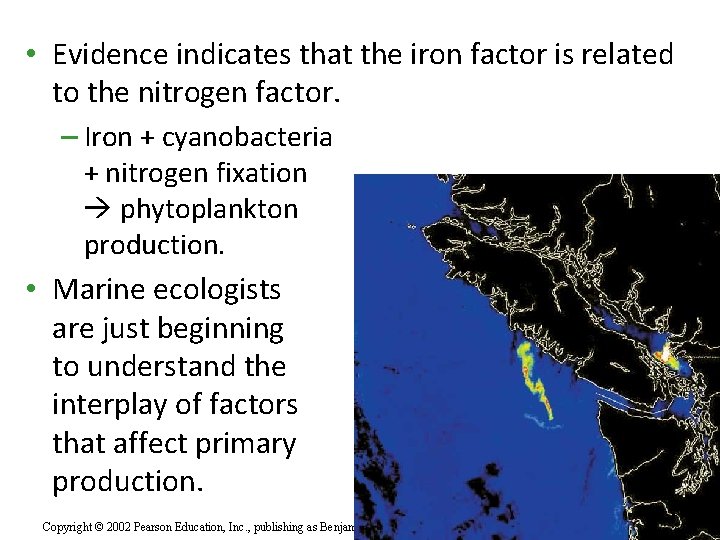
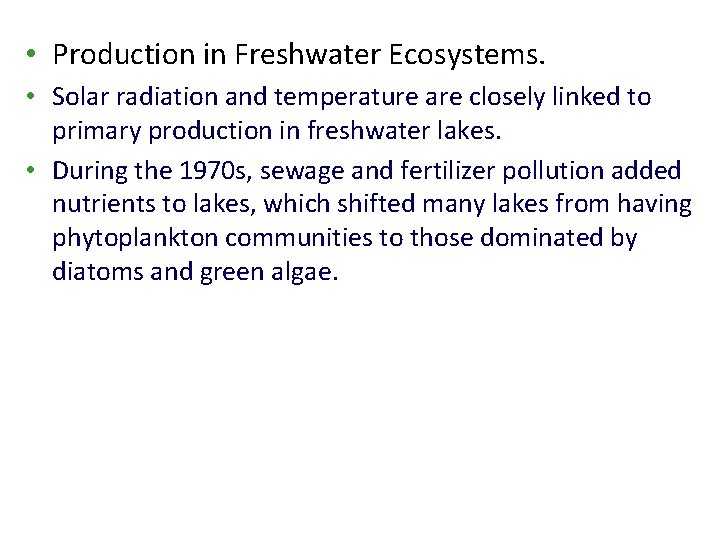
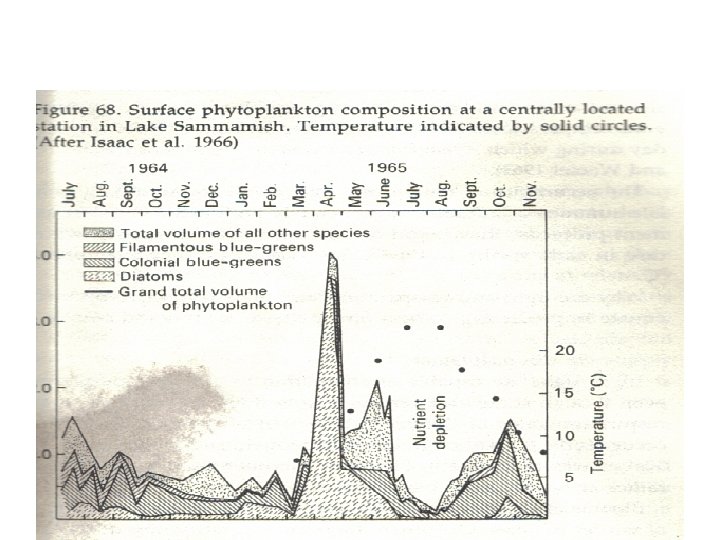
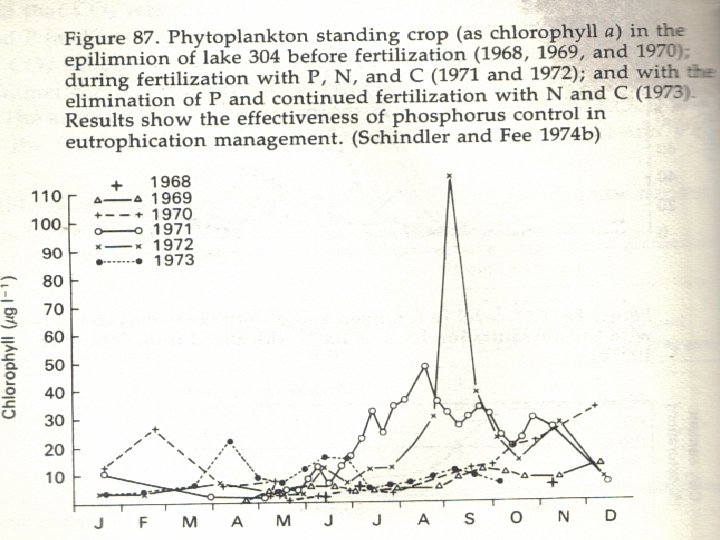
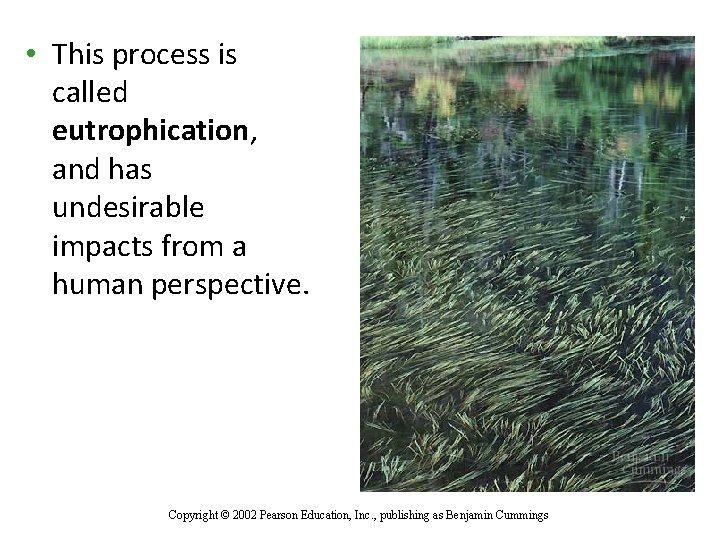
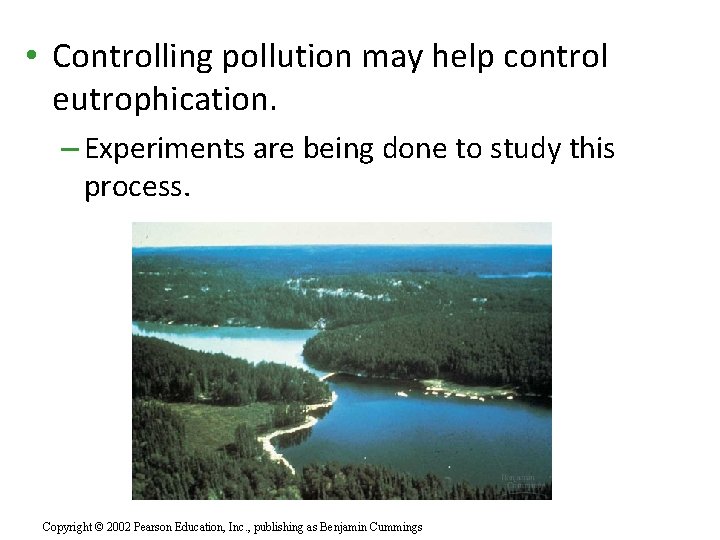
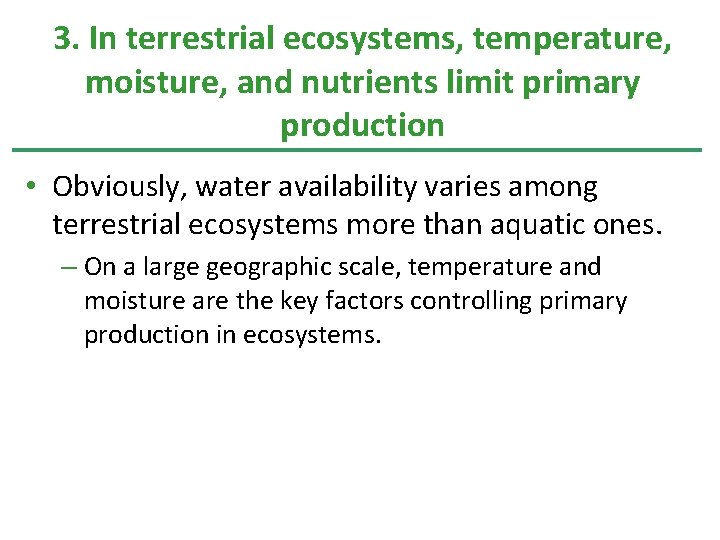
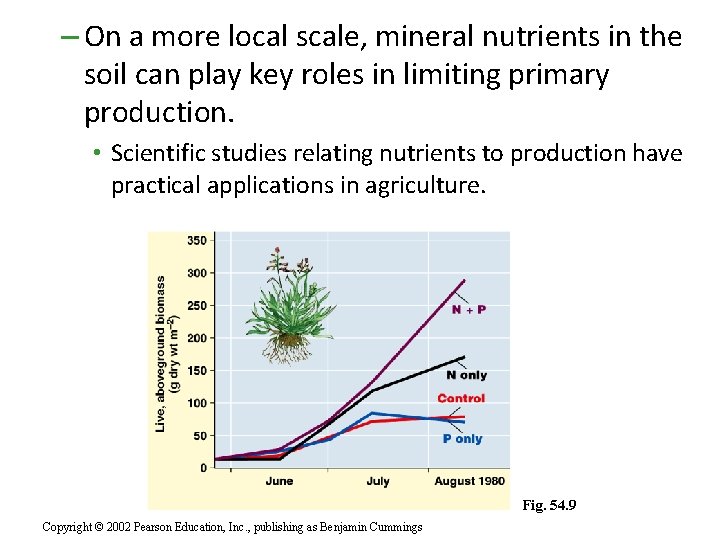
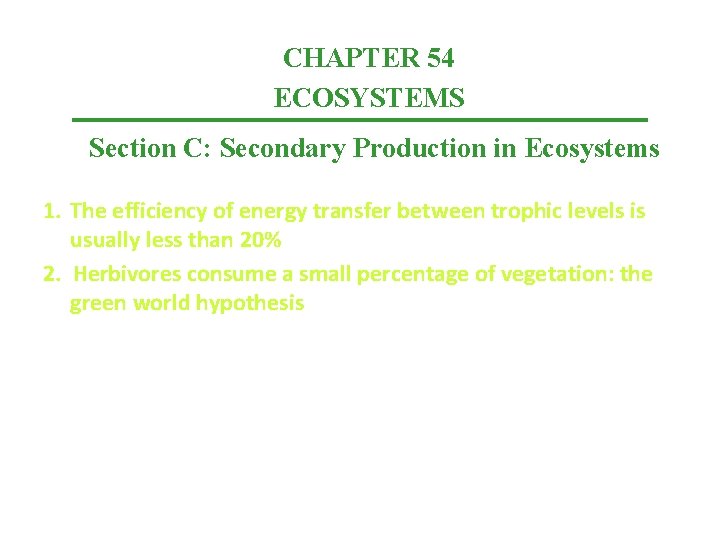
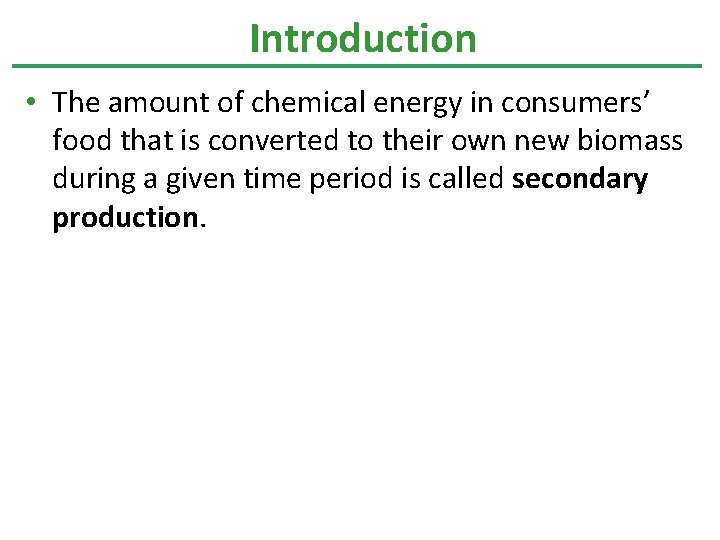
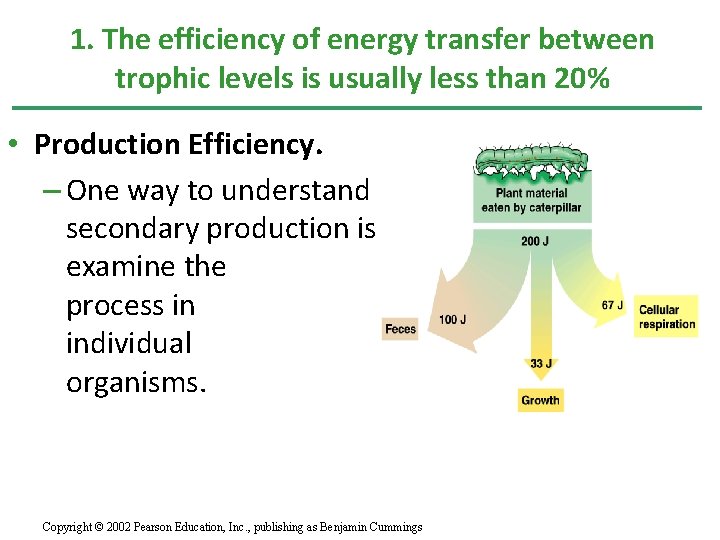
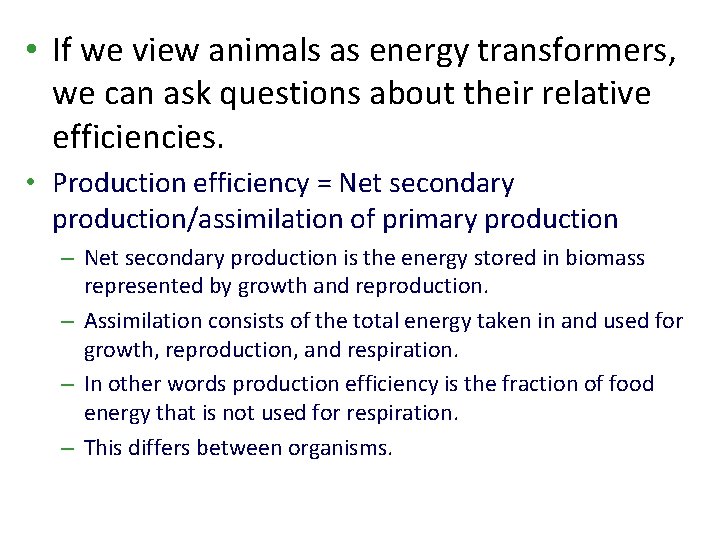
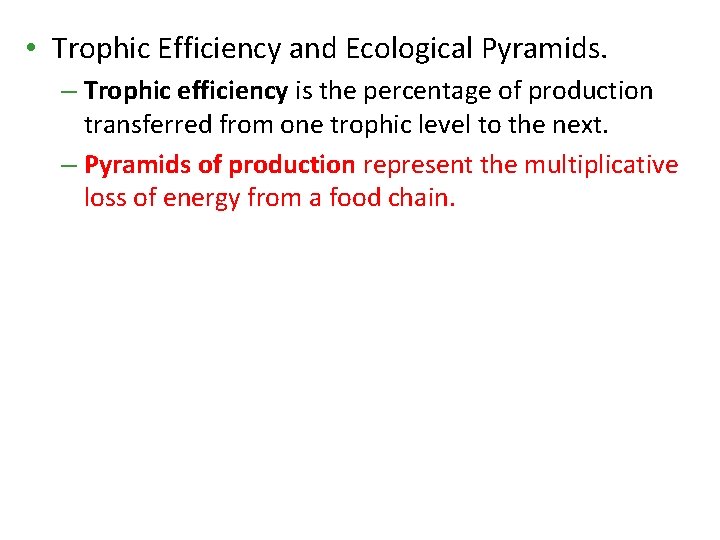
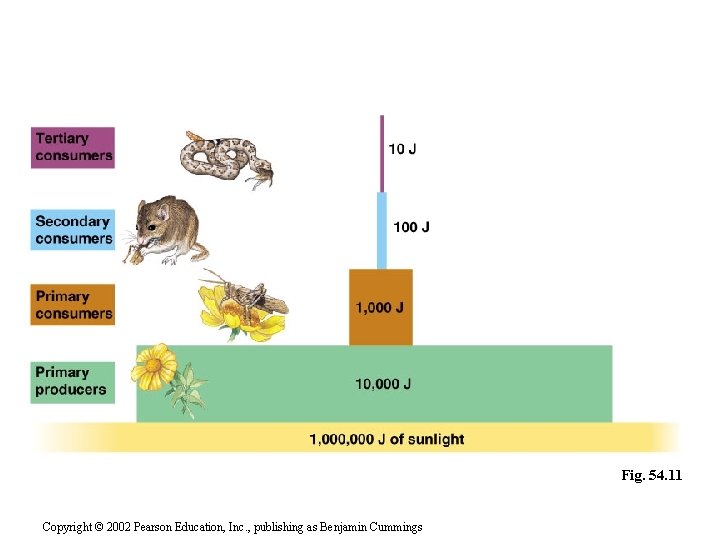
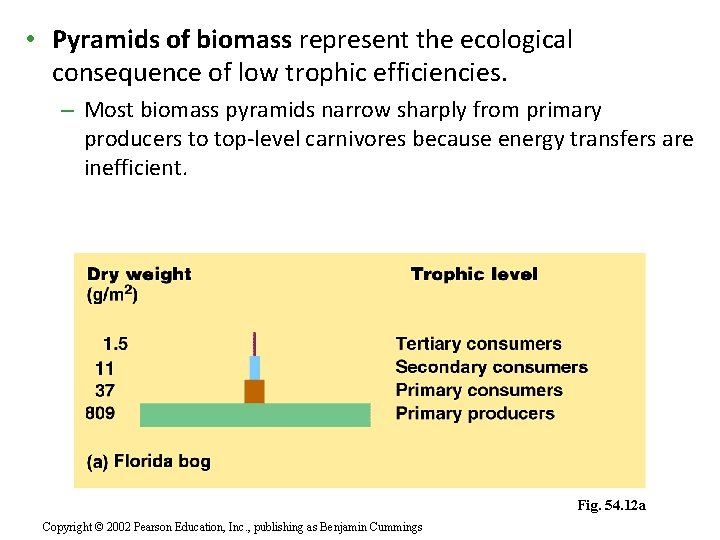
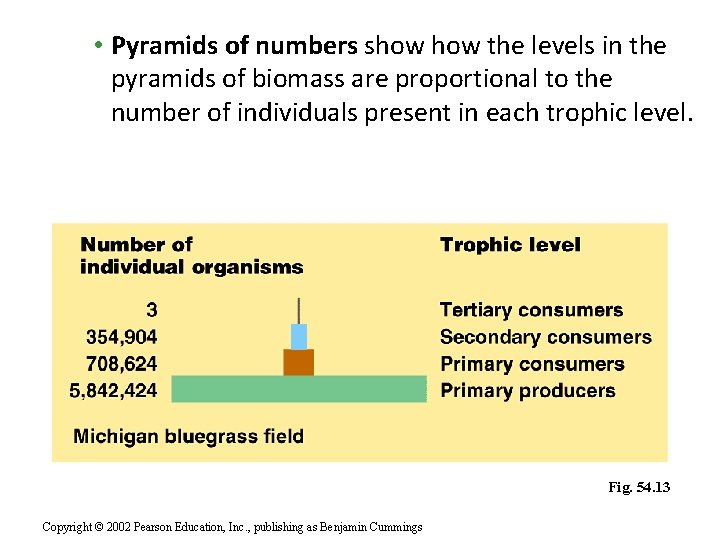
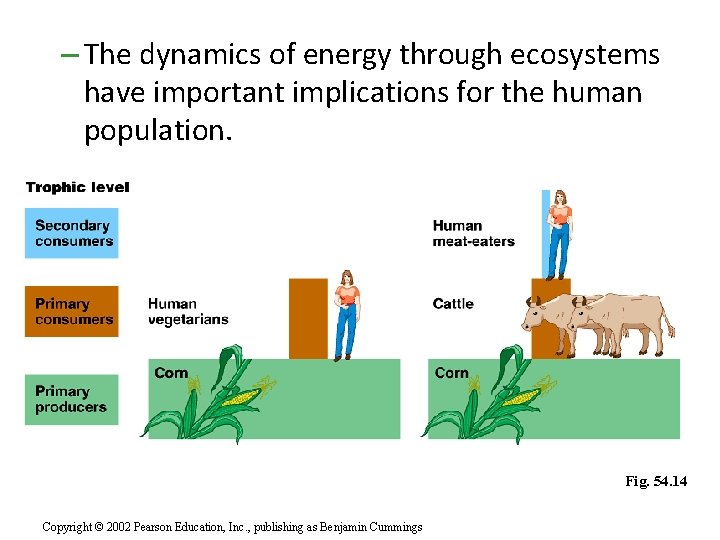
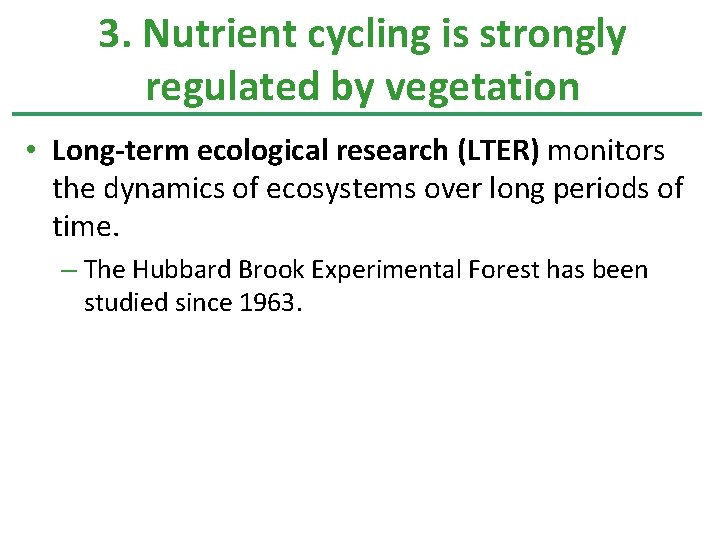
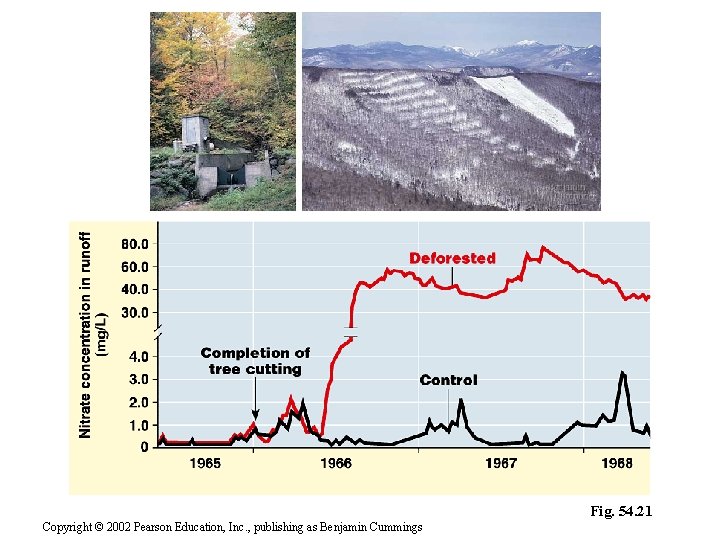
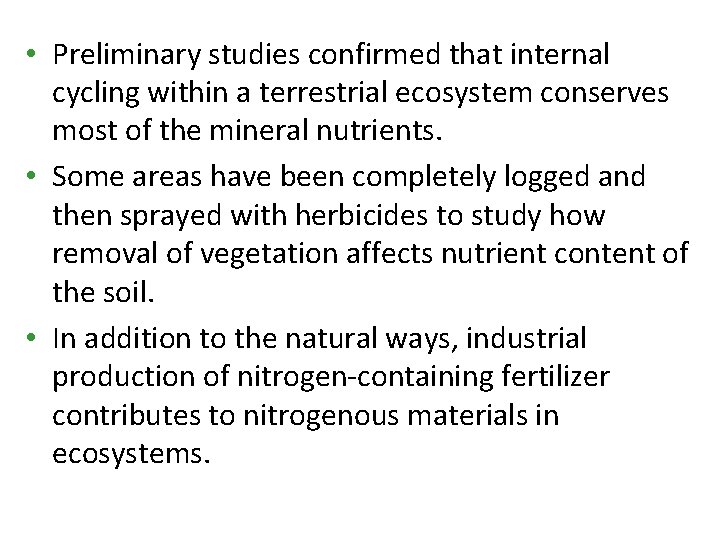
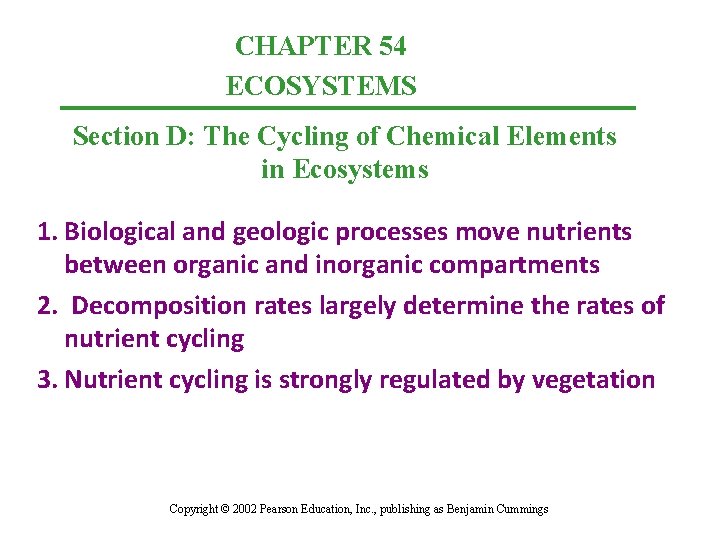
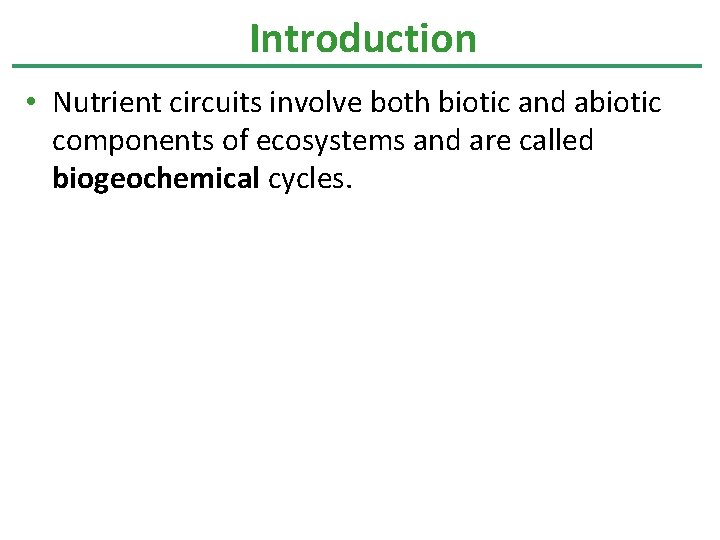
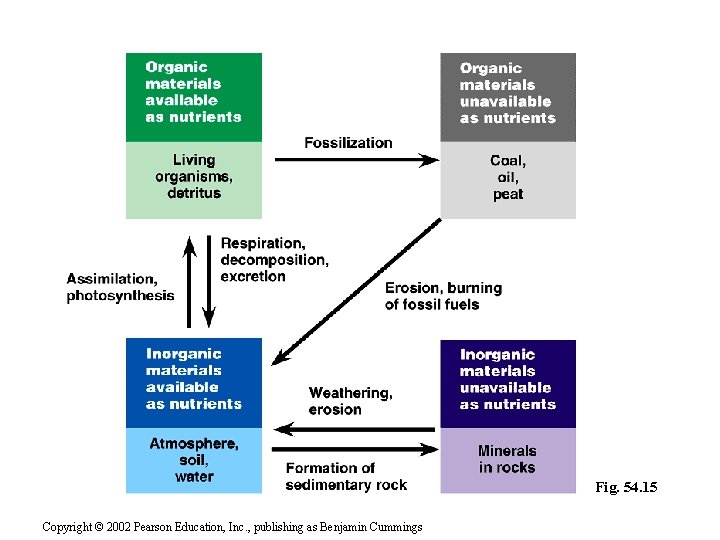
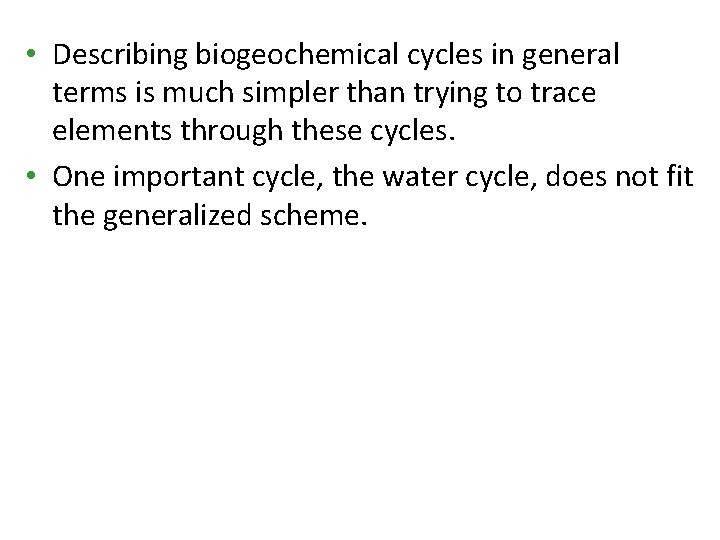
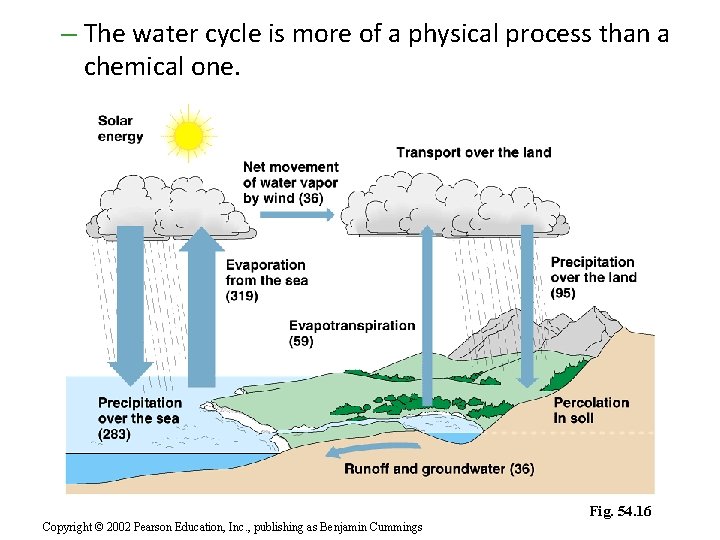
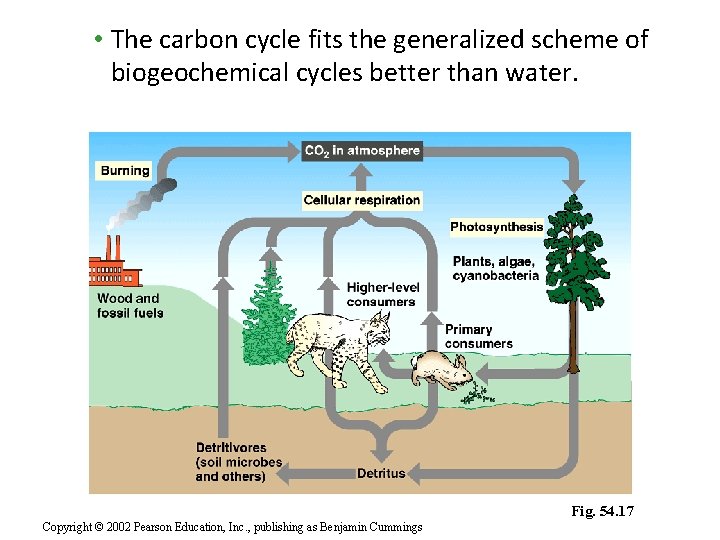
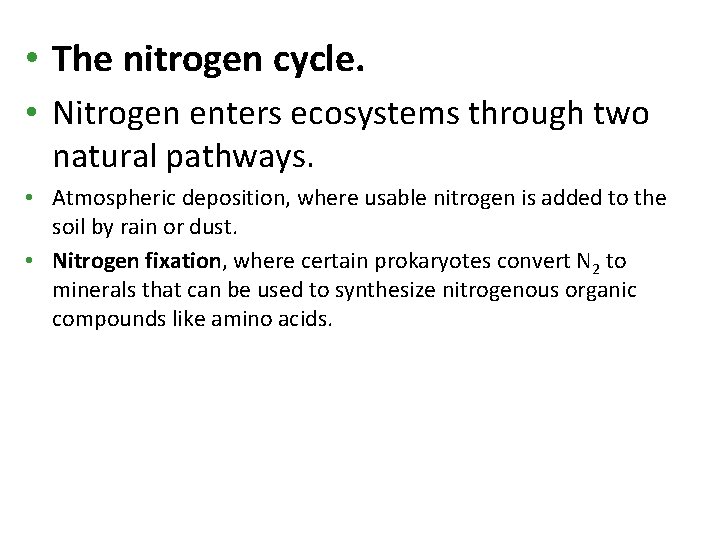
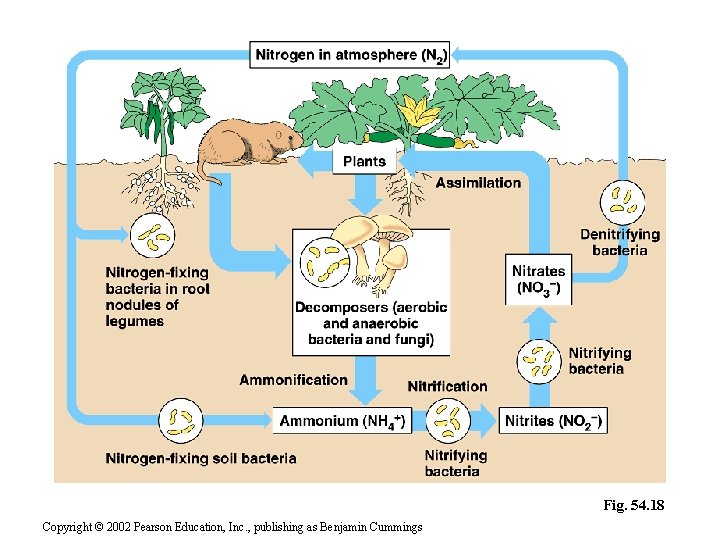
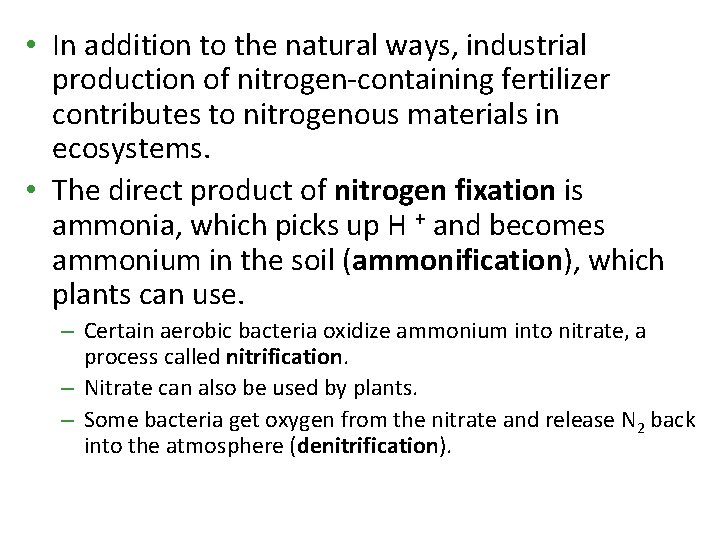
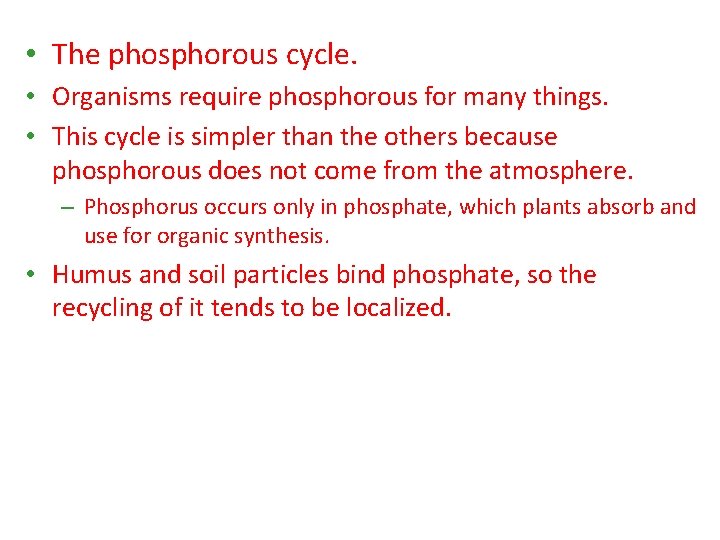
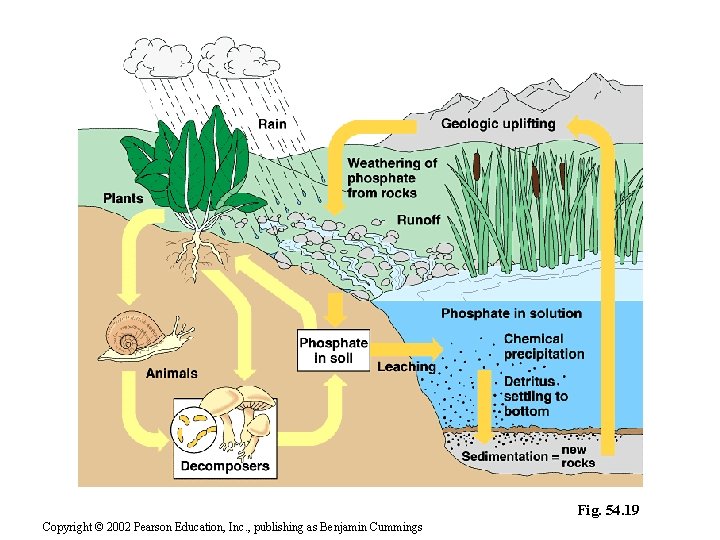
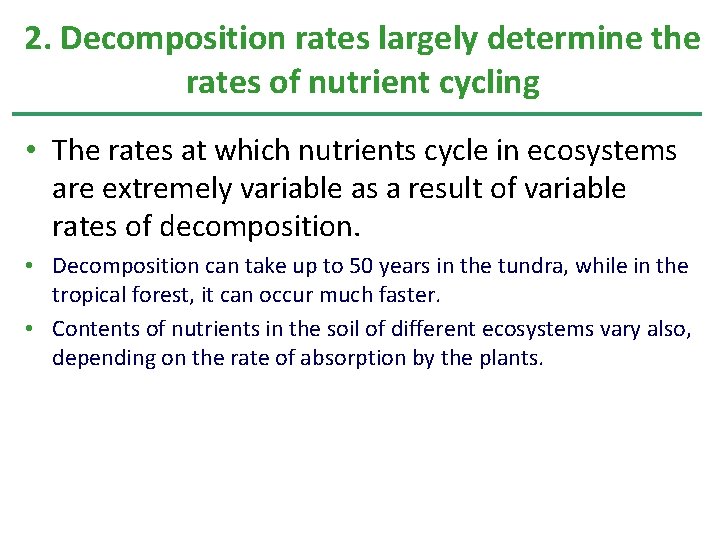
- Slides: 115
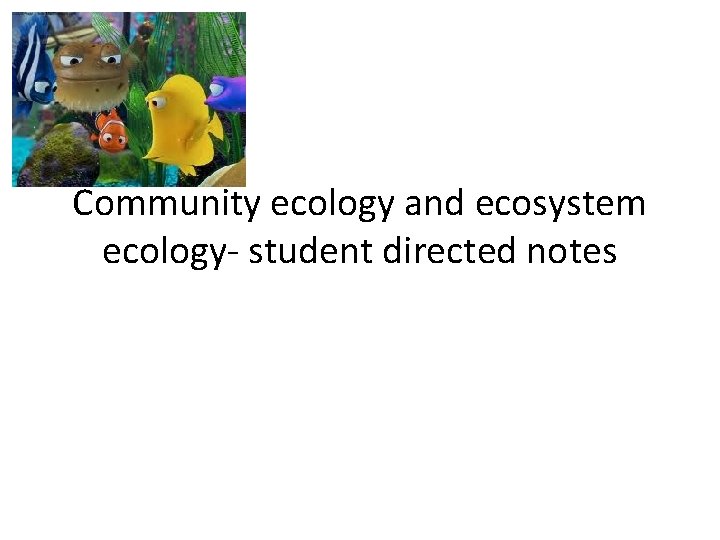
Community ecology and ecosystem ecology- student directed notes
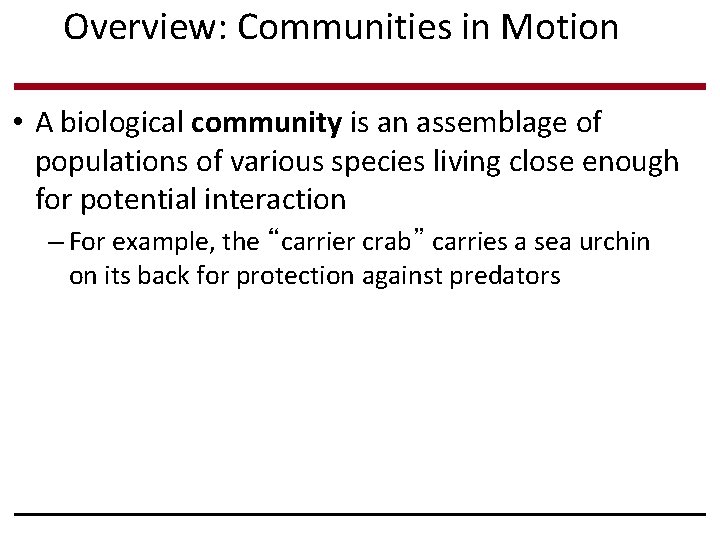
Overview: Communities in Motion • A biological community is an assemblage of populations of various species living close enough for potential interaction – For example, the “carrier crab” carries a sea urchin on its back for protection against predators
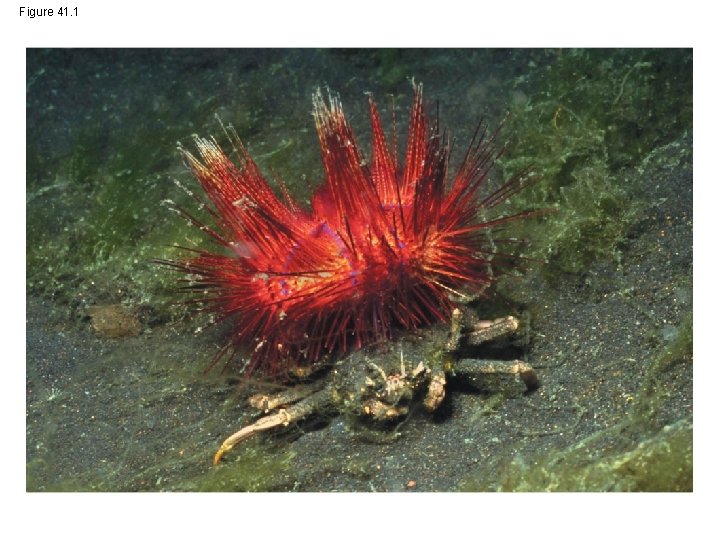
Figure 41. 1
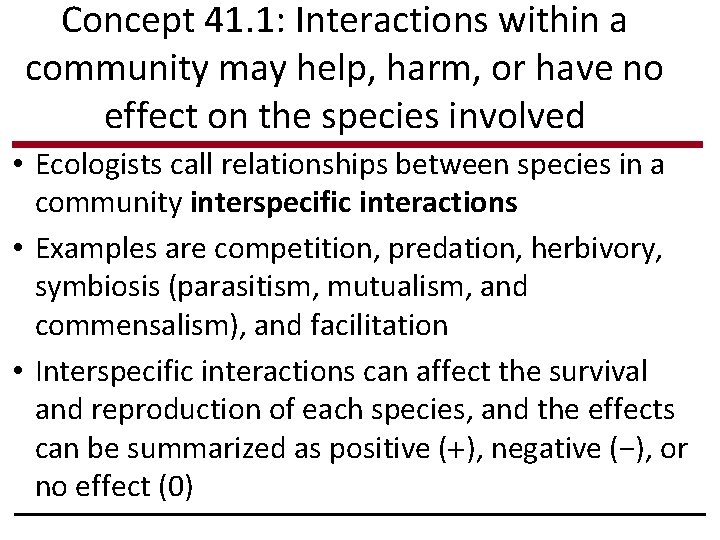
Concept 41. 1: Interactions within a community may help, harm, or have no effect on the species involved • Ecologists call relationships between species in a community interspecific interactions • Examples are competition, predation, herbivory, symbiosis (parasitism, mutualism, and commensalism), and facilitation • Interspecific interactions can affect the survival and reproduction of each species, and the effects can be summarized as positive ( ), negative (−), or no effect (0)
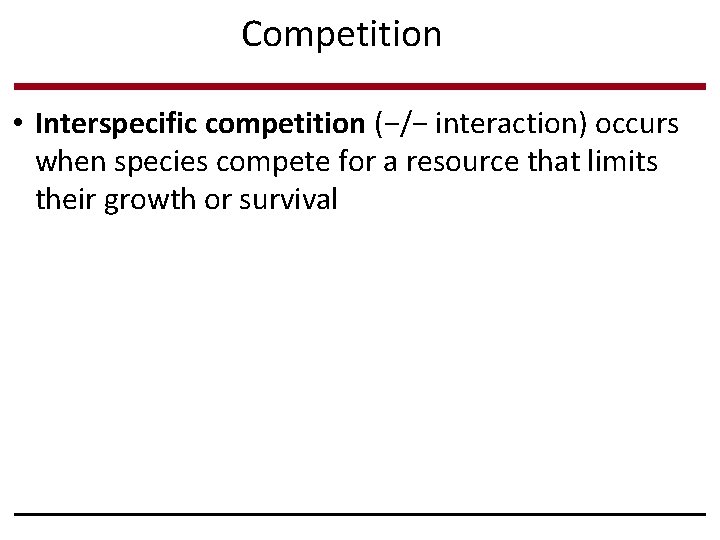
Competition • Interspecific competition (−/− interaction) occurs when species compete for a resource that limits their growth or survival
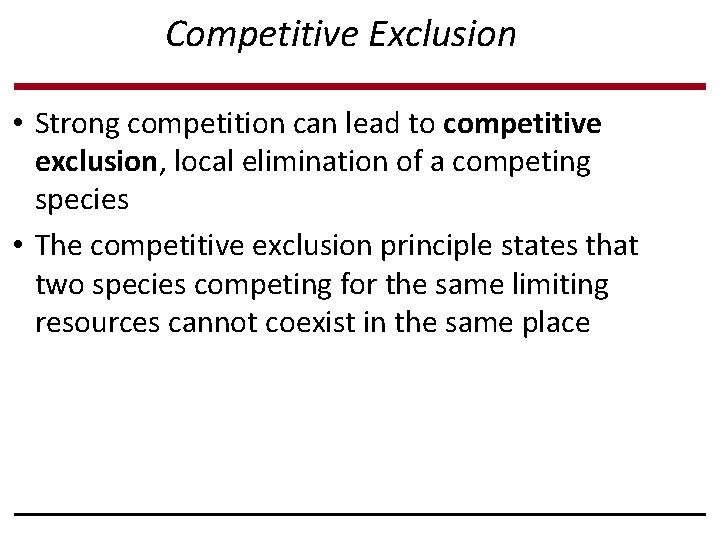
Competitive Exclusion • Strong competition can lead to competitive exclusion, local elimination of a competing species • The competitive exclusion principle states that two species competing for the same limiting resources cannot coexist in the same place
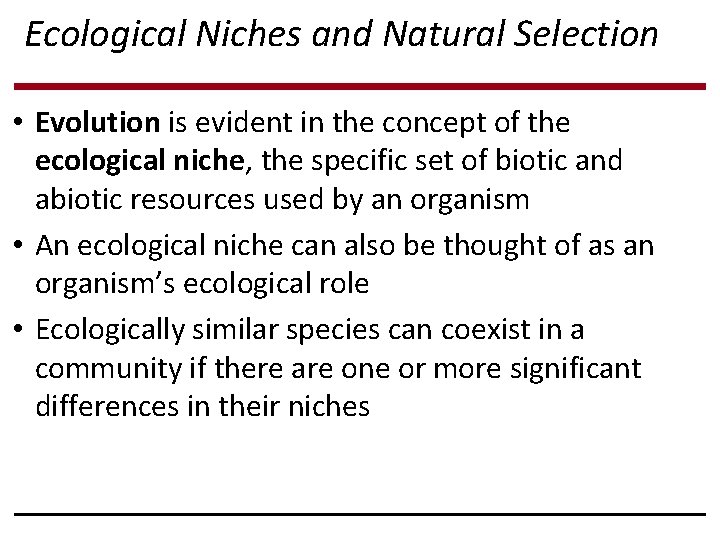
Ecological Niches and Natural Selection • Evolution is evident in the concept of the ecological niche, the specific set of biotic and abiotic resources used by an organism • An ecological niche can also be thought of as an organism’s ecological role • Ecologically similar species can coexist in a community if there are one or more significant differences in their niches
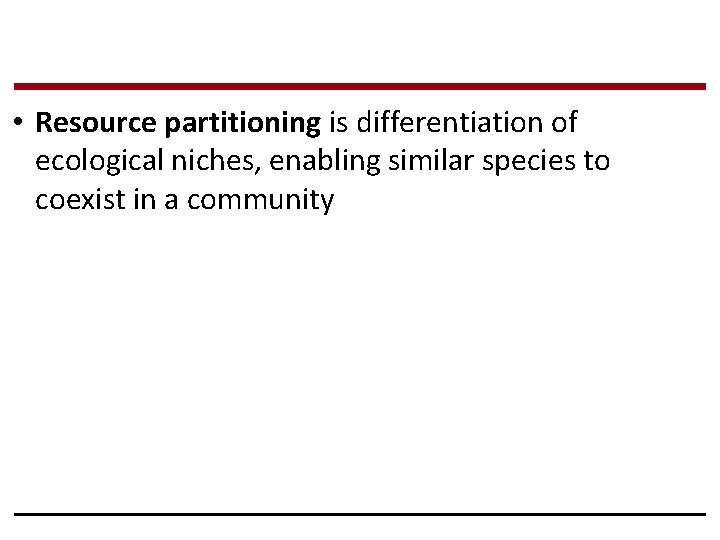
• Resource partitioning is differentiation of ecological niches, enabling similar species to coexist in a community
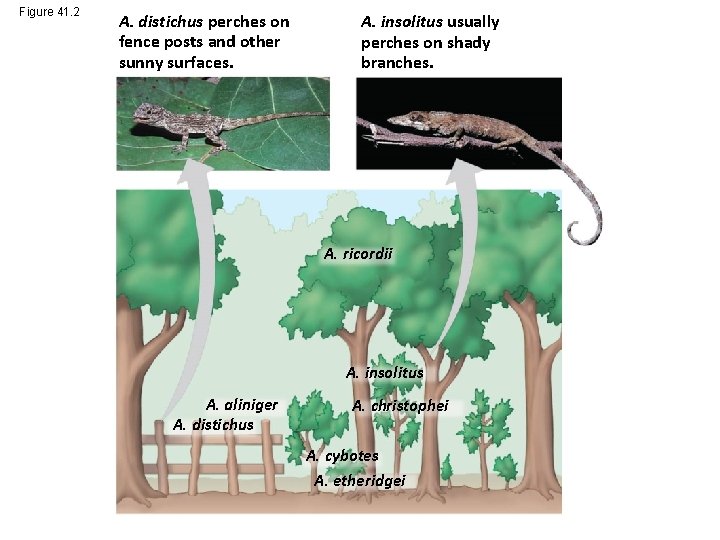
Figure 41. 2 A. distichus perches on fence posts and other sunny surfaces. A. insolitus usually perches on shady branches. A. ricordii A. insolitus A. aliniger A. distichus A. christophei A. cybotes A. etheridgei
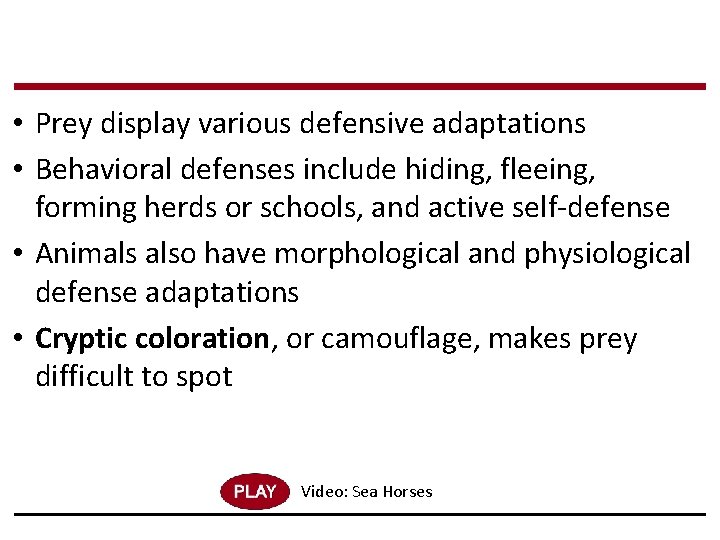
• Prey display various defensive adaptations • Behavioral defenses include hiding, fleeing, forming herds or schools, and active self-defense • Animals also have morphological and physiological defense adaptations • Cryptic coloration, or camouflage, makes prey difficult to spot Video: Sea Horses
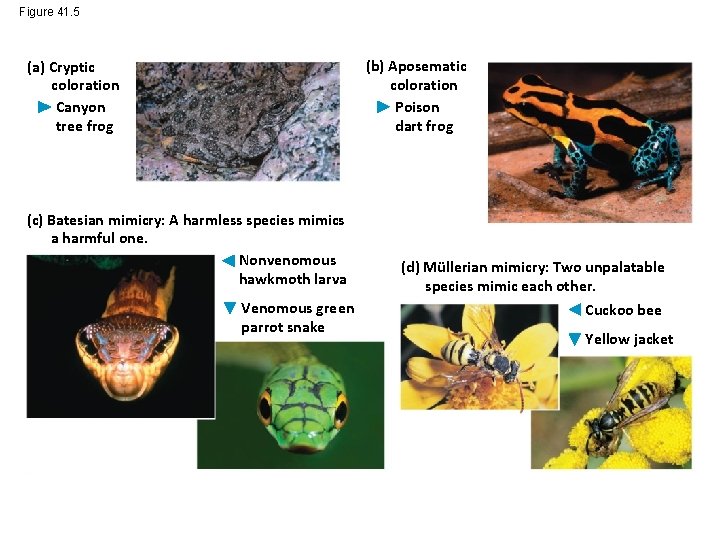
Figure 41. 5 (b) Aposematic coloration Poison dart frog (a) Cryptic coloration Canyon tree frog (c) Batesian mimicry: A harmless species mimics a harmful one. Nonvenomous hawkmoth larva Venomous green parrot snake (d) Müllerian mimicry: Two unpalatable species mimic each other. Cuckoo bee Yellow jacket
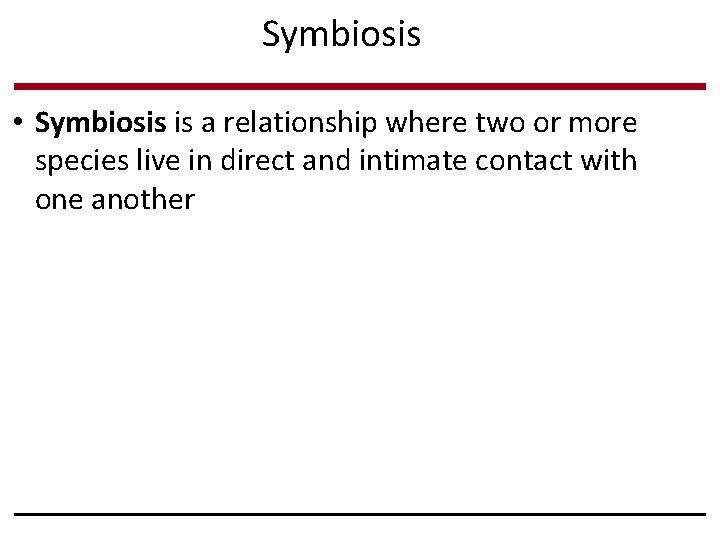
Symbiosis • Symbiosis is a relationship where two or more species live in direct and intimate contact with one another
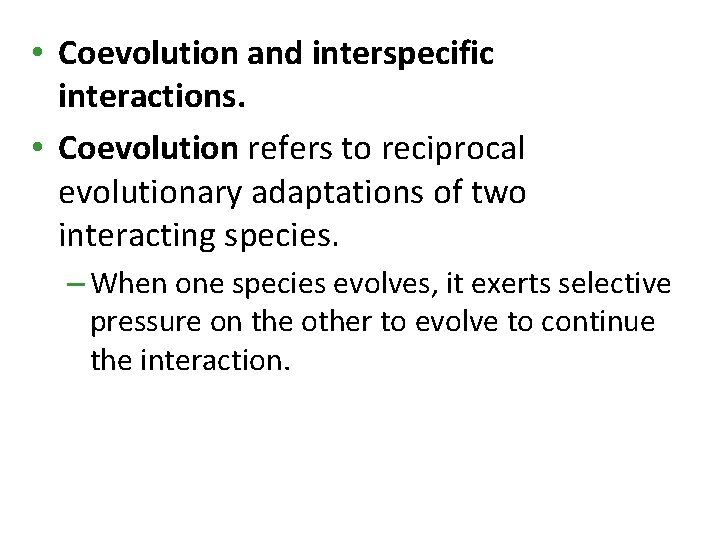
• Coevolution and interspecific interactions. • Coevolution refers to reciprocal evolutionary adaptations of two interacting species. – When one species evolves, it exerts selective pressure on the other to evolve to continue the interaction.
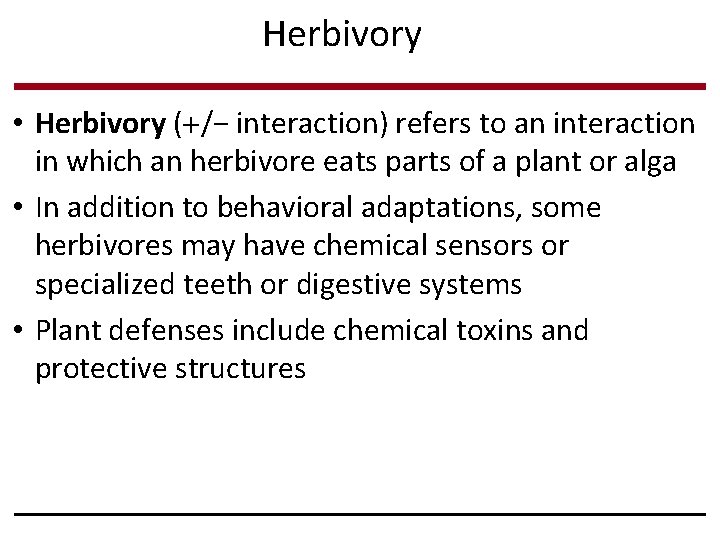
Herbivory • Herbivory ( /− interaction) refers to an interaction in which an herbivore eats parts of a plant or alga • In addition to behavioral adaptations, some herbivores may have chemical sensors or specialized teeth or digestive systems • Plant defenses include chemical toxins and protective structures
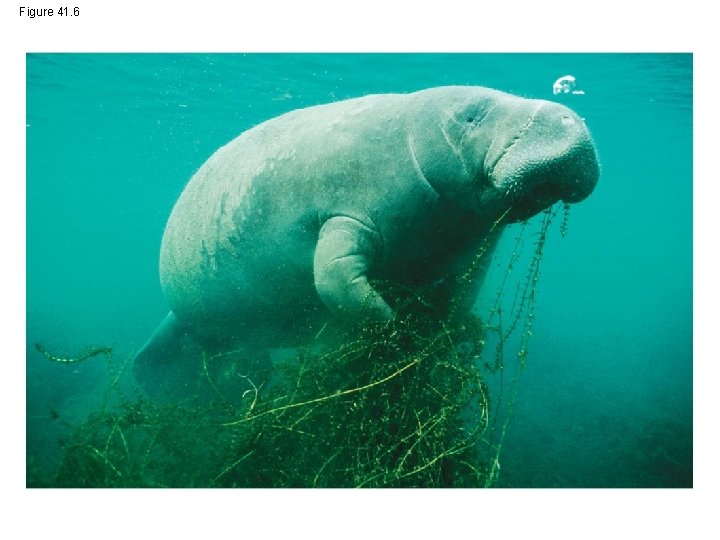
Figure 41. 6
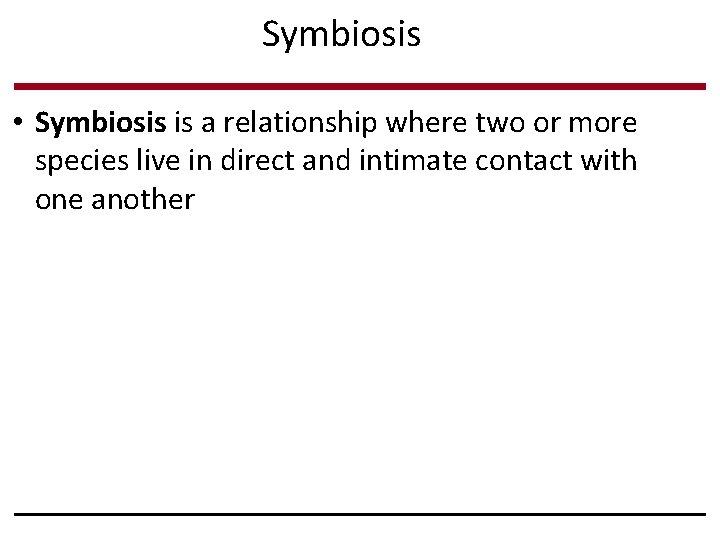
Symbiosis • Symbiosis is a relationship where two or more species live in direct and intimate contact with one another
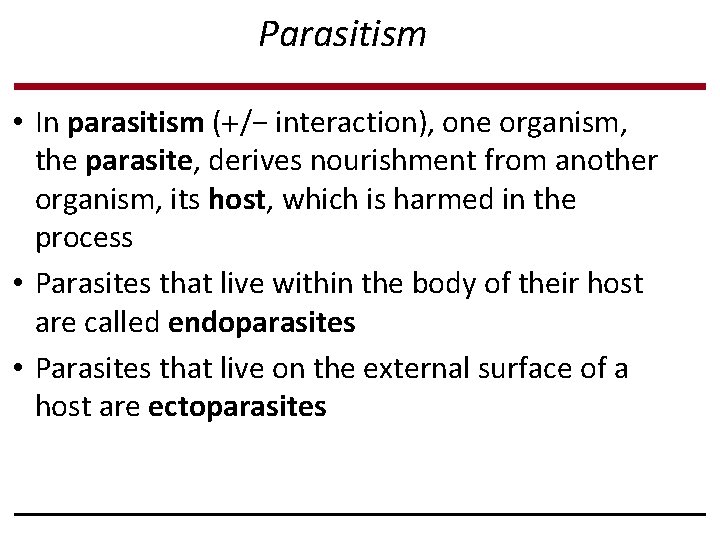
Parasitism • In parasitism ( /− interaction), one organism, the parasite, derives nourishment from another organism, its host, which is harmed in the process • Parasites that live within the body of their host are called endoparasites • Parasites that live on the external surface of a host are ectoparasites
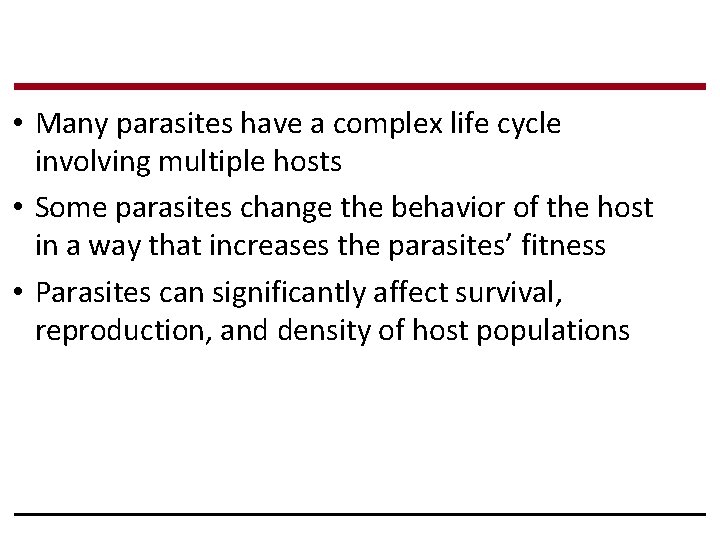
• Many parasites have a complex life cycle involving multiple hosts • Some parasites change the behavior of the host in a way that increases the parasites’ fitness • Parasites can significantly affect survival, reproduction, and density of host populations
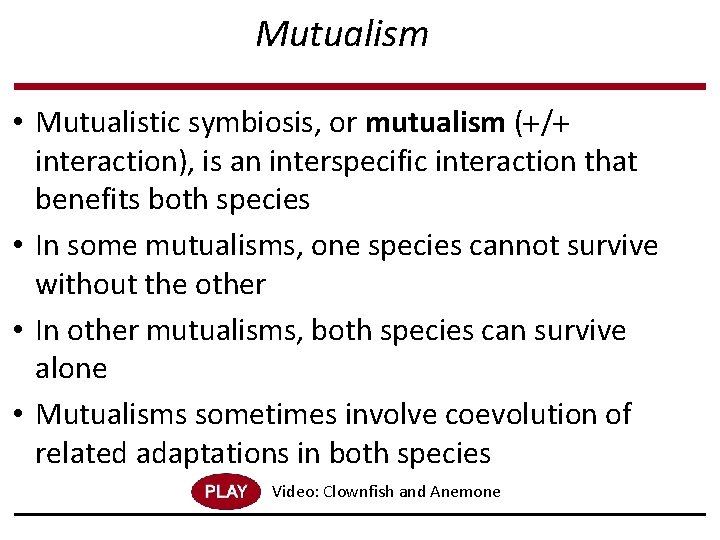
Mutualism • Mutualistic symbiosis, or mutualism ( / interaction), is an interspecific interaction that benefits both species • In some mutualisms, one species cannot survive without the other • In other mutualisms, both species can survive alone • Mutualisms sometimes involve coevolution of related adaptations in both species Video: Clownfish and Anemone
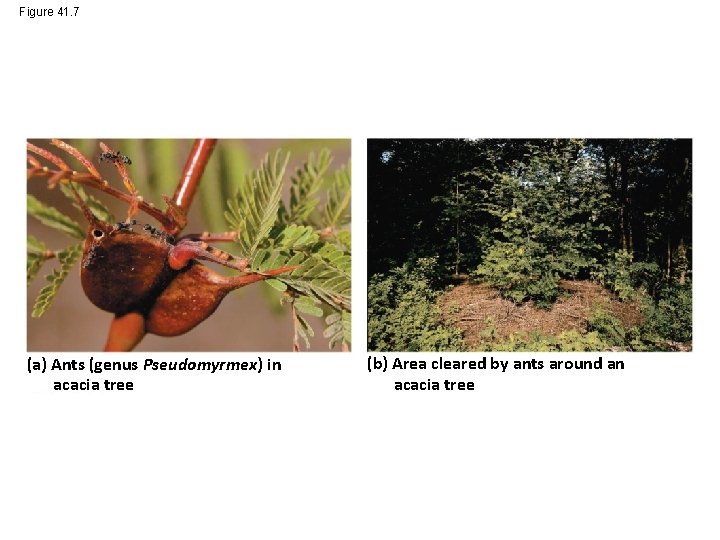
Figure 41. 7 (a) Ants (genus Pseudomyrmex) in acacia tree (b) Area cleared by ants around an acacia tree
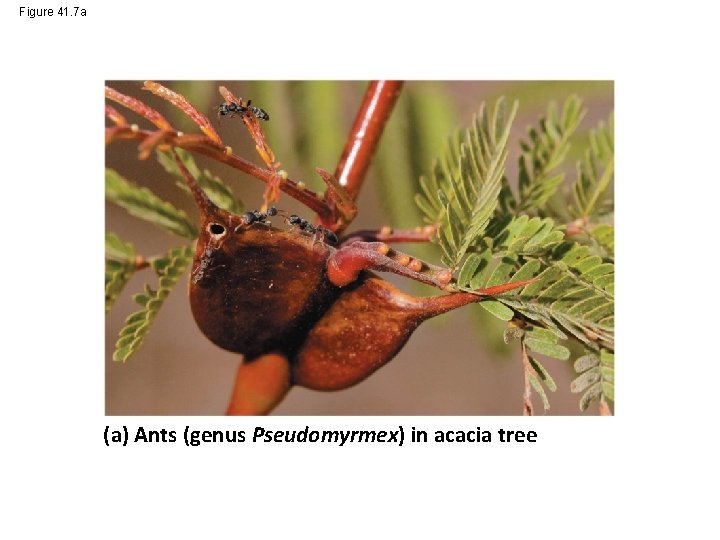
Figure 41. 7 a (a) Ants (genus Pseudomyrmex) in acacia tree
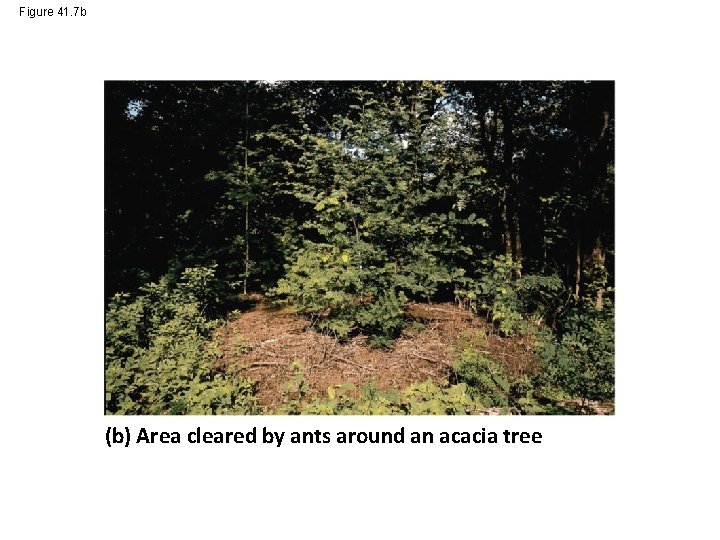
Figure 41. 7 b (b) Area cleared by ants around an acacia tree
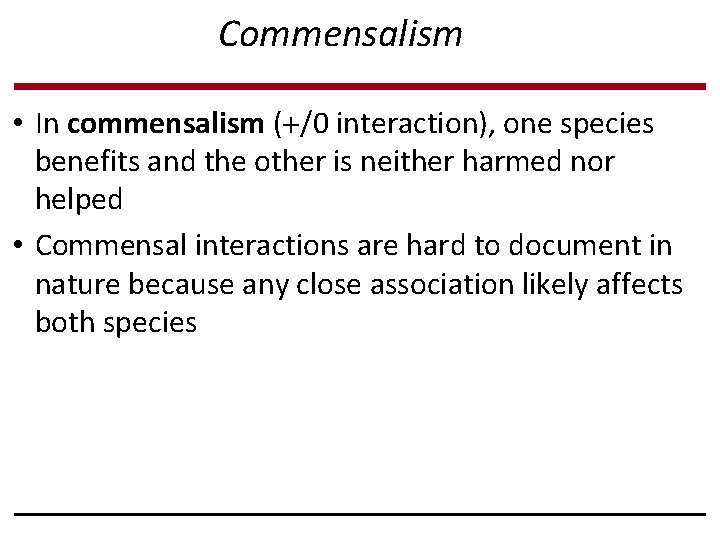
Commensalism • In commensalism ( /0 interaction), one species benefits and the other is neither harmed nor helped • Commensal interactions are hard to document in nature because any close association likely affects both species
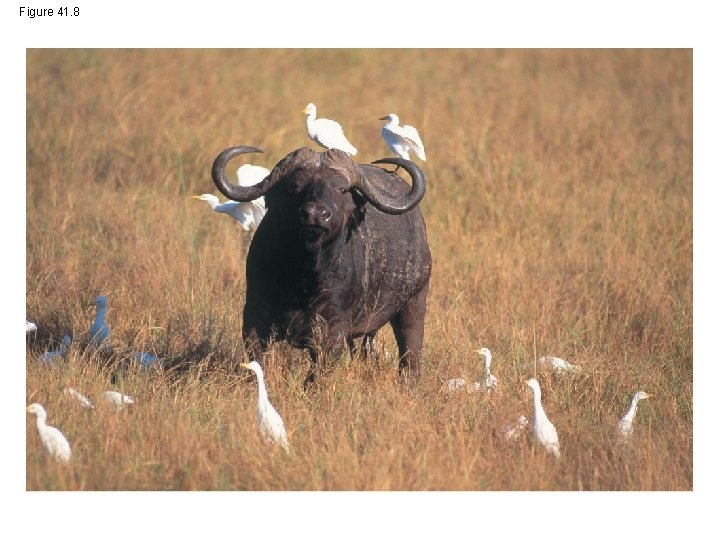
Figure 41. 8
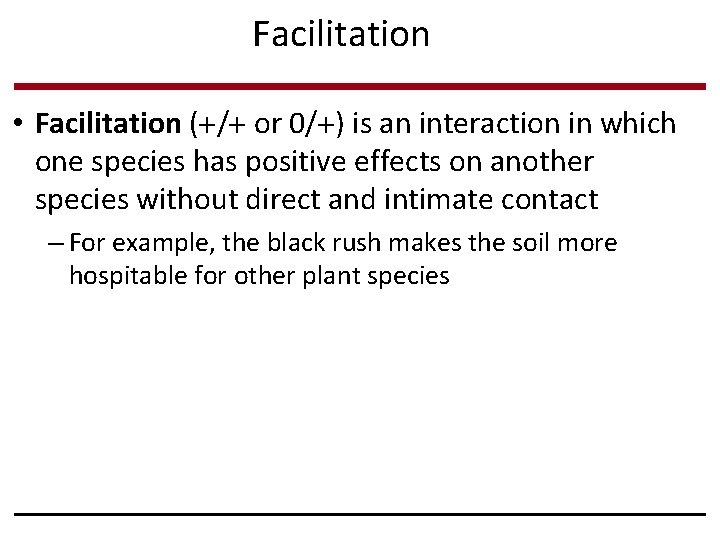
Facilitation • Facilitation ( / or 0/ ) is an interaction in which one species has positive effects on another species without direct and intimate contact – For example, the black rush makes the soil more hospitable for other plant species
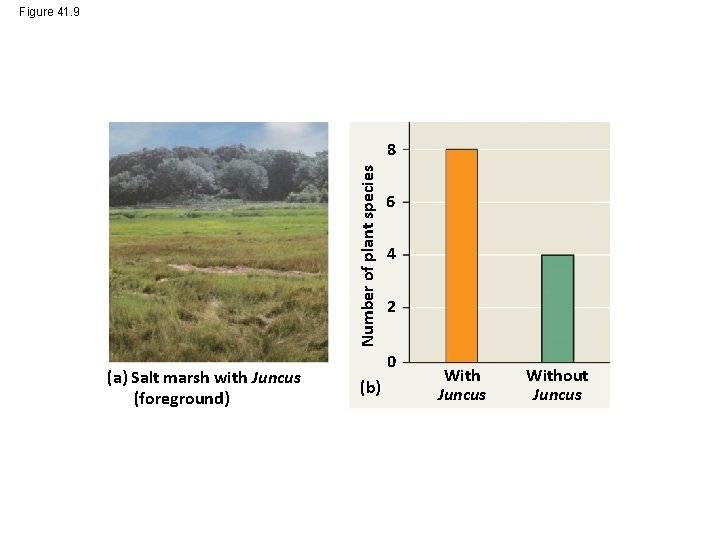
Figure 41. 9 Number of plant species 8 (a) Salt marsh with Juncus (foreground) 6 4 2 0 (b) With Juncus Without Juncus
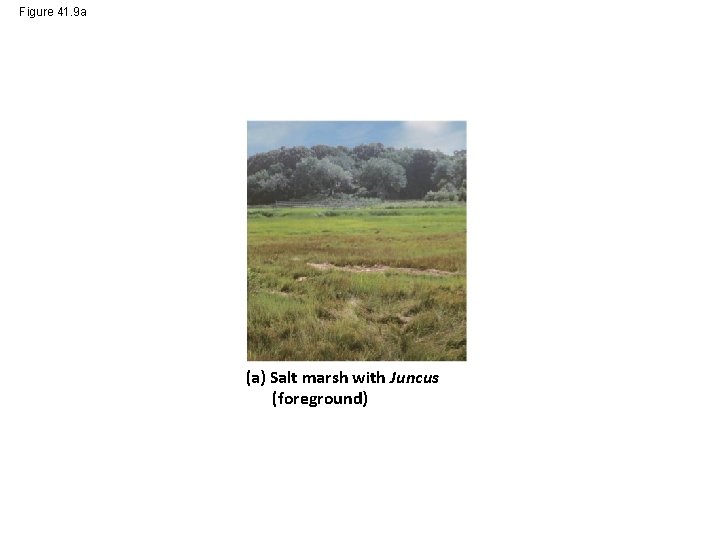
Figure 41. 9 a (a) Salt marsh with Juncus (foreground)
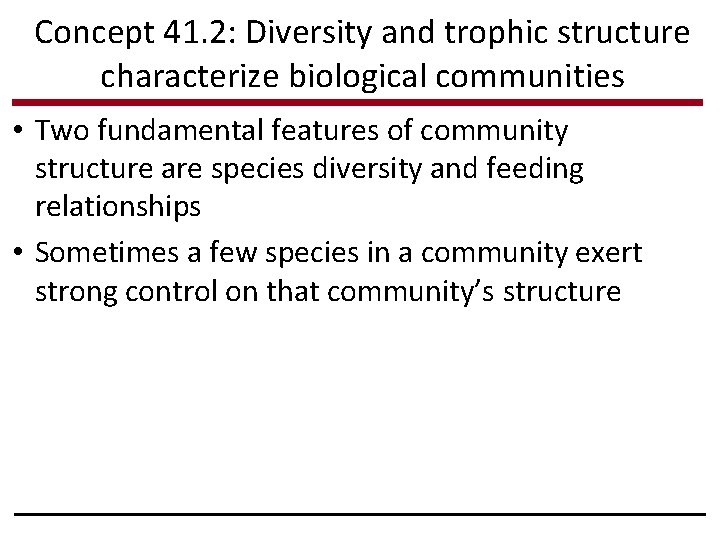
Concept 41. 2: Diversity and trophic structure characterize biological communities • Two fundamental features of community structure are species diversity and feeding relationships • Sometimes a few species in a community exert strong control on that community’s structure
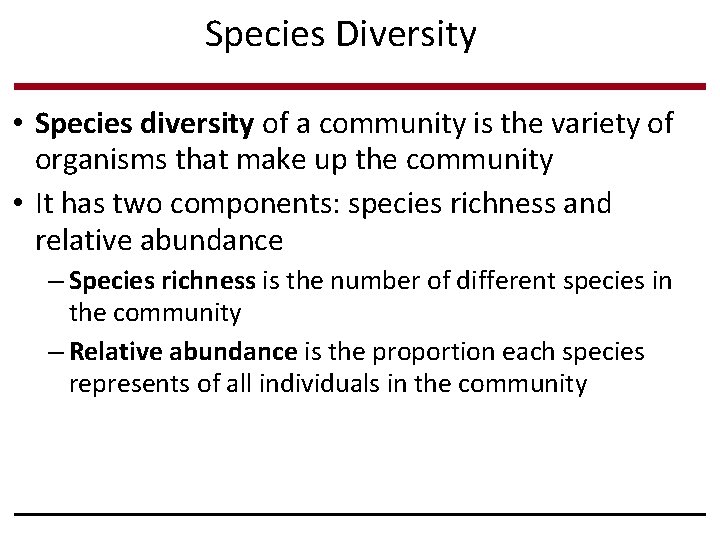
Species Diversity • Species diversity of a community is the variety of organisms that make up the community • It has two components: species richness and relative abundance – Species richness is the number of different species in the community – Relative abundance is the proportion each species represents of all individuals in the community
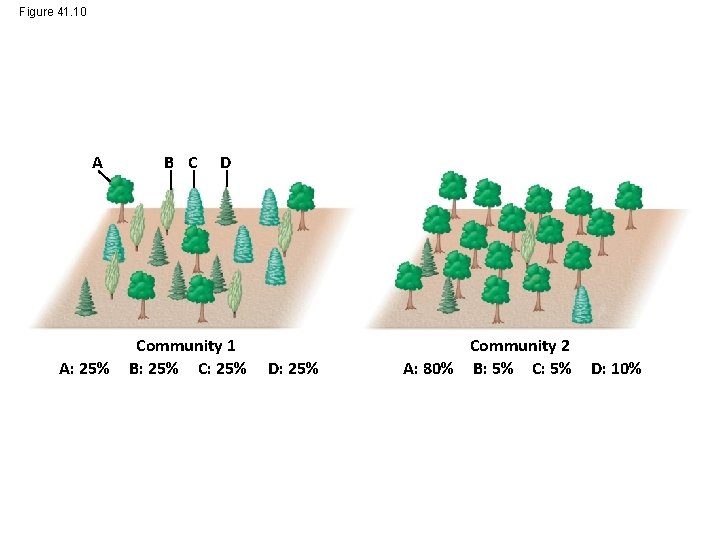
Figure 41. 10 A A: 25% B C D Community 1 B: 25% C: 25% D: 25% Community 2 A: 80% B: 5% C: 5% D: 10%
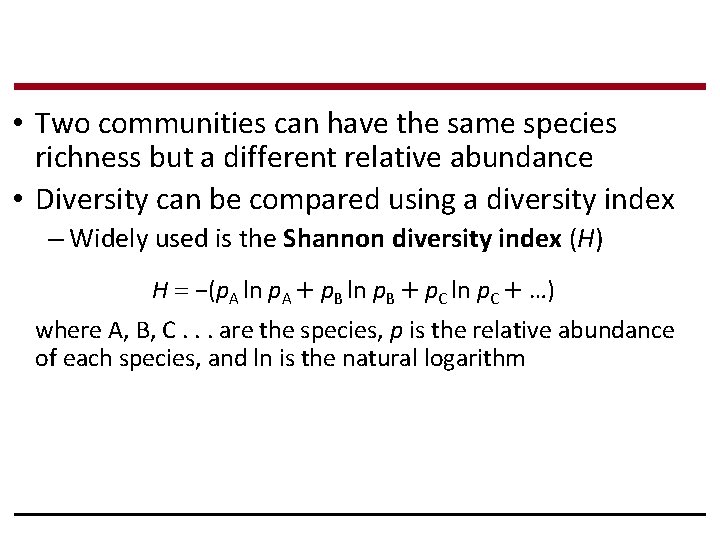
• Two communities can have the same species richness but a different relative abundance • Diversity can be compared using a diversity index – Widely used is the Shannon diversity index (H) H −(p. A ln p. A p. B ln p. B p. C ln p. C …) where A, B, C. . . are the species, p is the relative abundance of each species, and ln is the natural logarithm
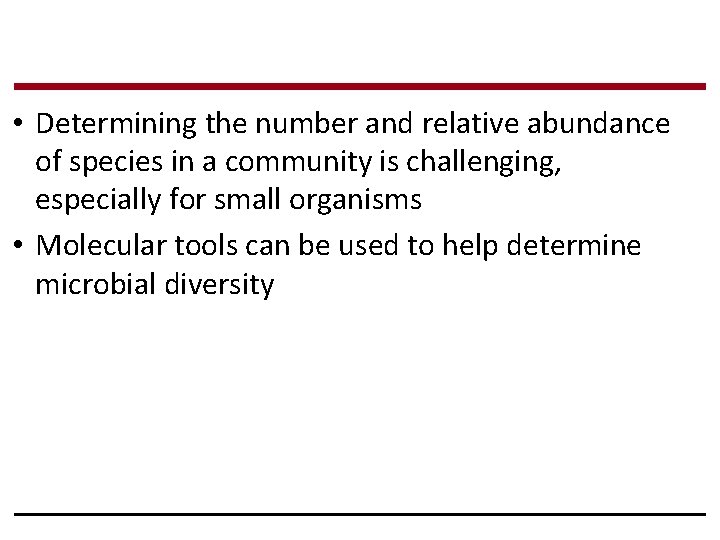
• Determining the number and relative abundance of species in a community is challenging, especially for small organisms • Molecular tools can be used to help determine microbial diversity
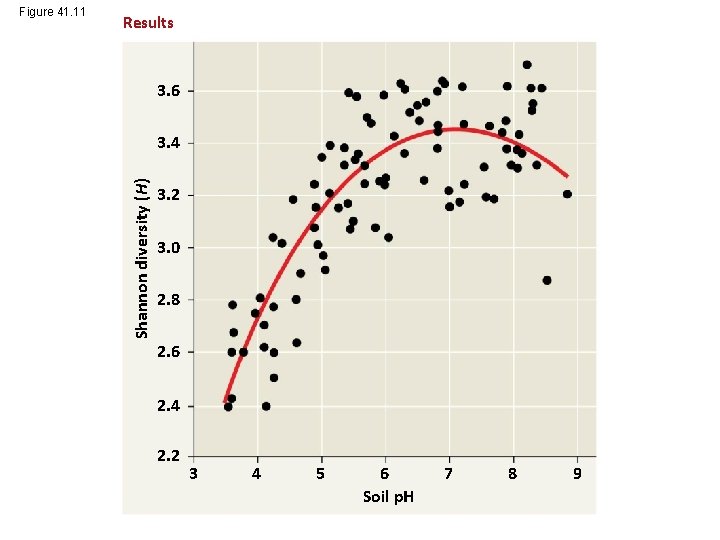
Results 3. 6 3. 4 Shannon diversity (H) Figure 41. 11 3. 2 3. 0 2. 8 2. 6 2. 4 2. 2 3 4 5 6 Soil p. H 7 8 9
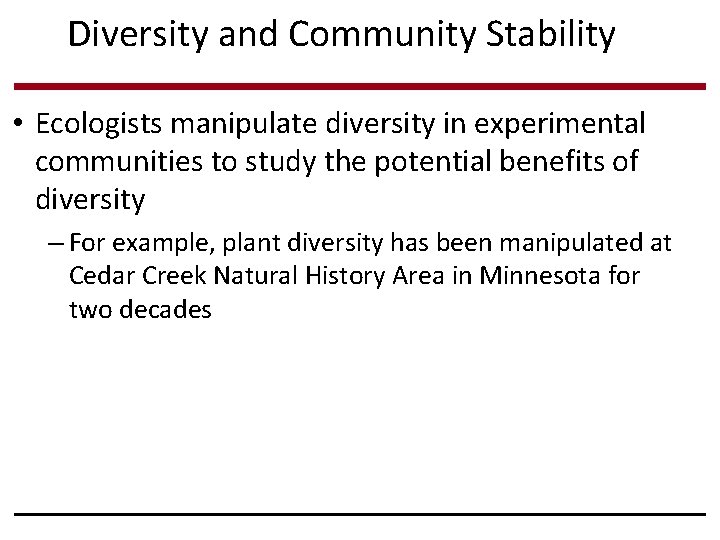
Diversity and Community Stability • Ecologists manipulate diversity in experimental communities to study the potential benefits of diversity – For example, plant diversity has been manipulated at Cedar Creek Natural History Area in Minnesota for two decades
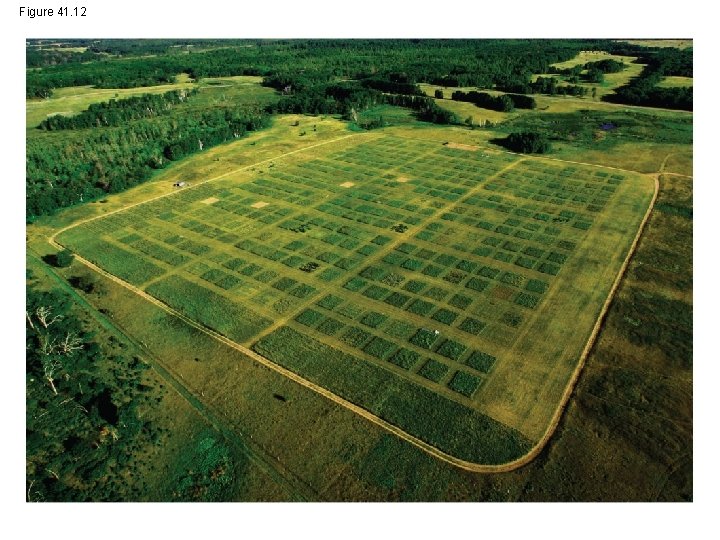
Figure 41. 12
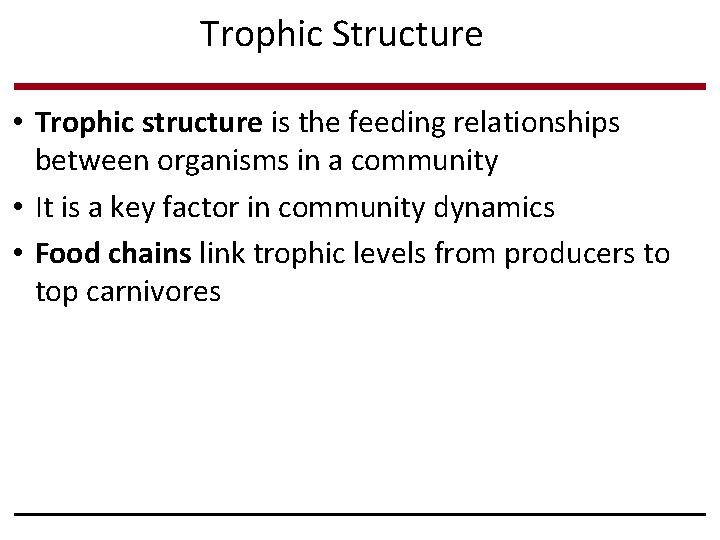
Trophic Structure • Trophic structure is the feeding relationships between organisms in a community • It is a key factor in community dynamics • Food chains link trophic levels from producers to top carnivores
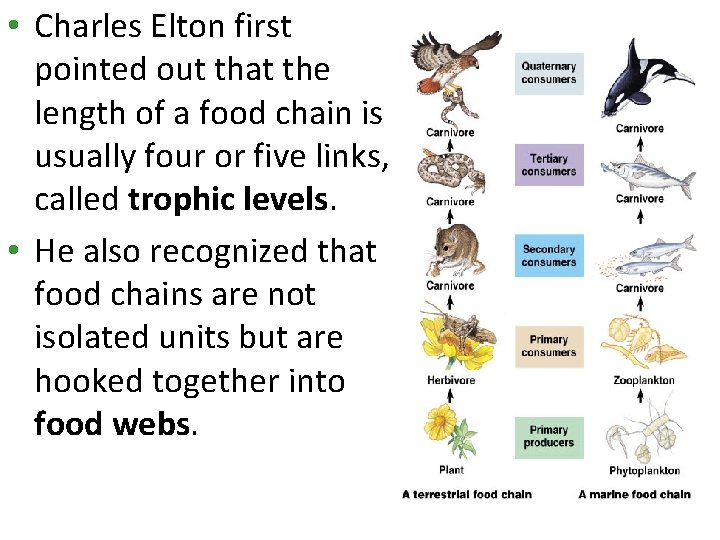
• Charles Elton first pointed out that the length of a food chain is usually four or five links, called trophic levels. • He also recognized that food chains are not isolated units but are hooked together into food webs.
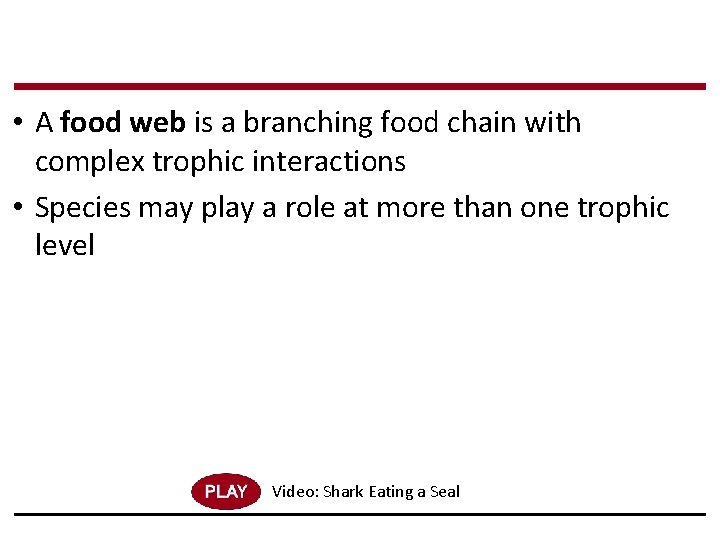
• A food web is a branching food chain with complex trophic interactions • Species may play a role at more than one trophic level Video: Shark Eating a Seal
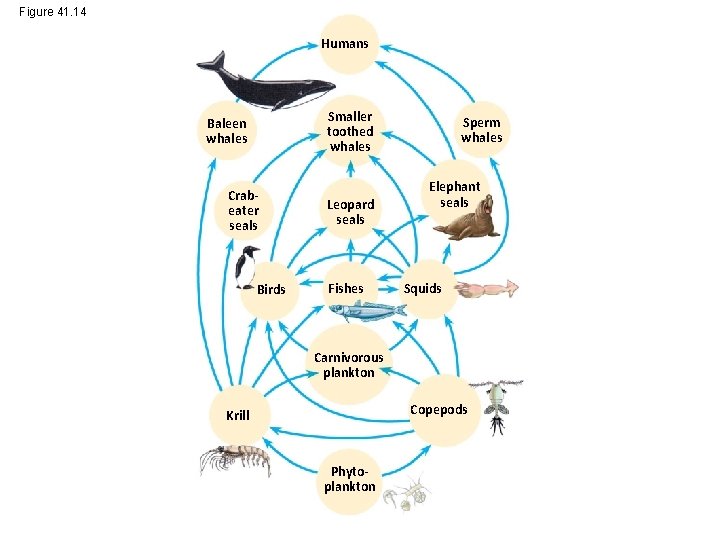
Figure 41. 14 Humans Smaller toothed whales Baleen whales Crabeater seals Birds Leopard seals Fishes Sperm whales Elephant seals Squids Carnivorous plankton Copepods Krill Phytoplankton
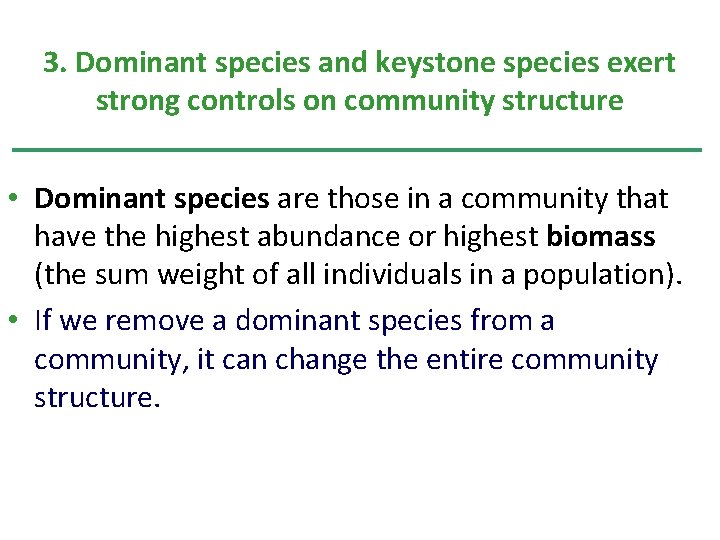
3. Dominant species and keystone species exert strong controls on community structure • Dominant species are those in a community that have the highest abundance or highest biomass (the sum weight of all individuals in a population). • If we remove a dominant species from a community, it can change the entire community structure.
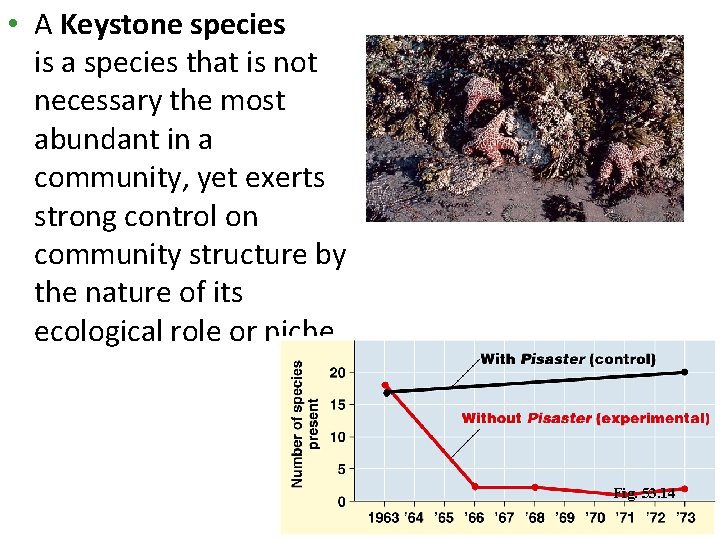
• A Keystone species is a species that is not necessary the most abundant in a community, yet exerts strong control on community structure by the nature of its ecological role or niche. Fig. 53. 14
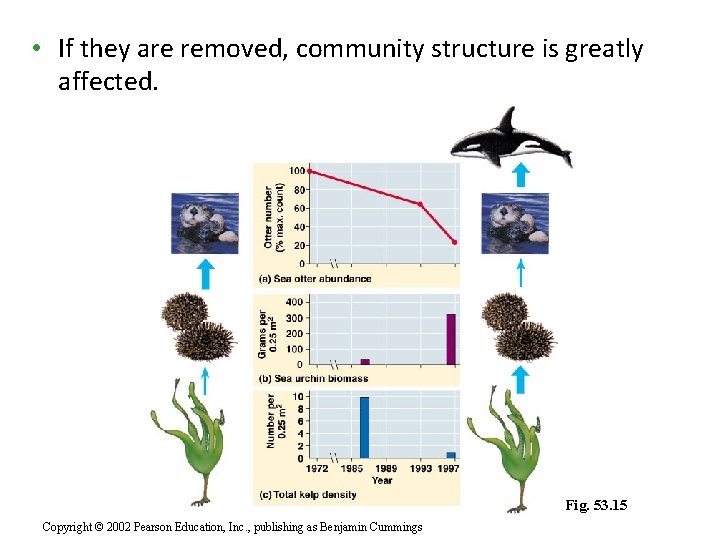
• If they are removed, community structure is greatly affected. Fig. 53. 15 Copyright © 2002 Pearson Education, Inc. , publishing as Benjamin Cummings
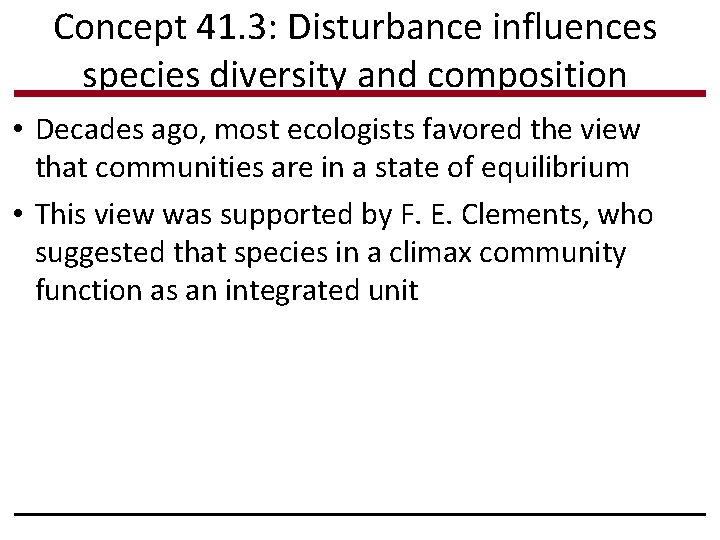
Concept 41. 3: Disturbance influences species diversity and composition • Decades ago, most ecologists favored the view that communities are in a state of equilibrium • This view was supported by F. E. Clements, who suggested that species in a climax community function as an integrated unit
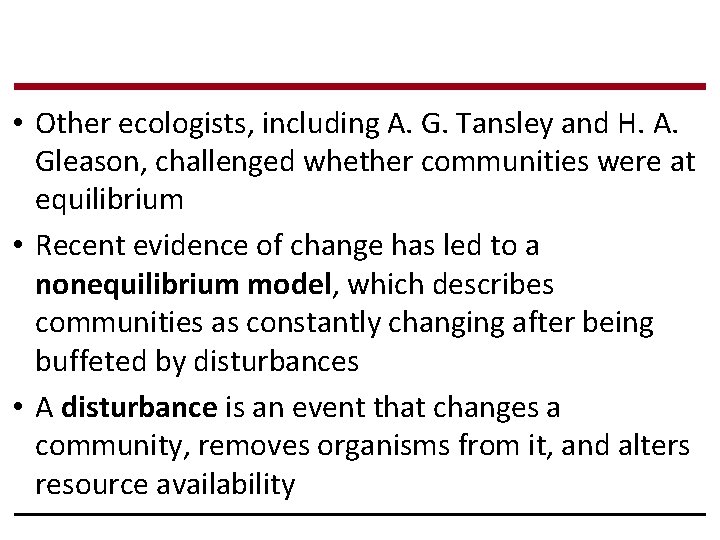
• Other ecologists, including A. G. Tansley and H. A. Gleason, challenged whether communities were at equilibrium • Recent evidence of change has led to a nonequilibrium model, which describes communities as constantly changing after being buffeted by disturbances • A disturbance is an event that changes a community, removes organisms from it, and alters resource availability
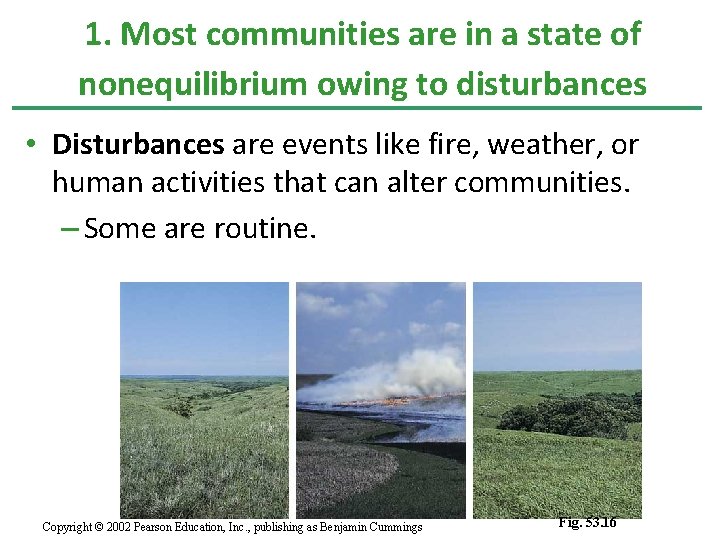
1. Most communities are in a state of nonequilibrium owing to disturbances • Disturbances are events like fire, weather, or human activities that can alter communities. – Some are routine. Copyright © 2002 Pearson Education, Inc. , publishing as Benjamin Cummings Fig. 53. 16
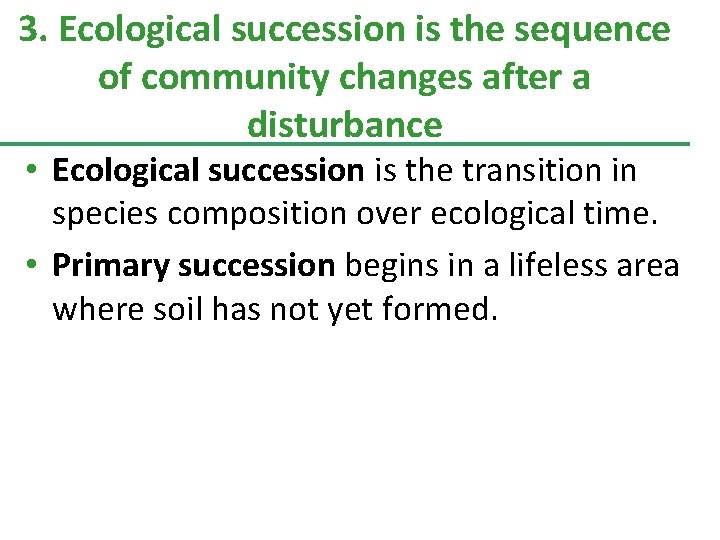
3. Ecological succession is the sequence of community changes after a disturbance • Ecological succession is the transition in species composition over ecological time. • Primary succession begins in a lifeless area where soil has not yet formed.
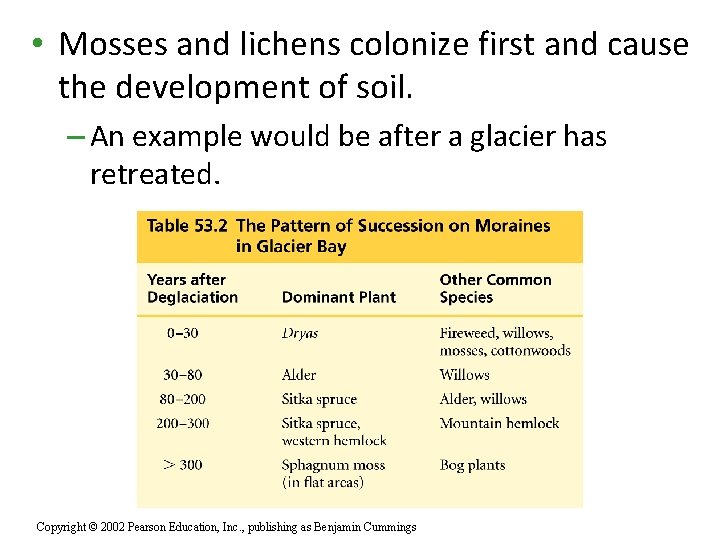
• Mosses and lichens colonize first and cause the development of soil. – An example would be after a glacier has retreated. Copyright © 2002 Pearson Education, Inc. , publishing as Benjamin Cummings
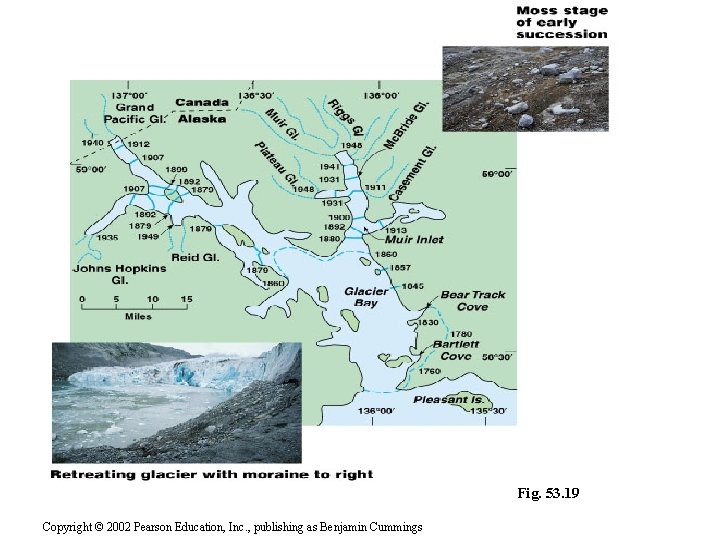
Fig. 53. 19 Copyright © 2002 Pearson Education, Inc. , publishing as Benjamin Cummings
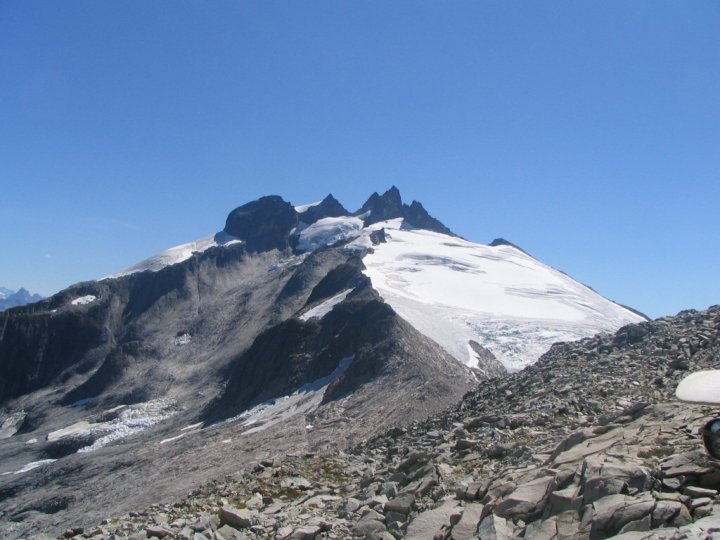
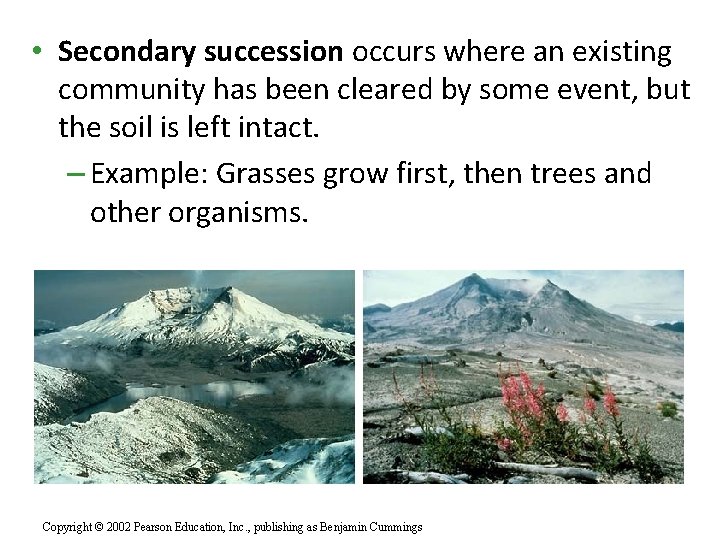
• Secondary succession occurs where an existing community has been cleared by some event, but the soil is left intact. – Example: Grasses grow first, then trees and other organisms. Copyright © 2002 Pearson Education, Inc. , publishing as Benjamin Cummings
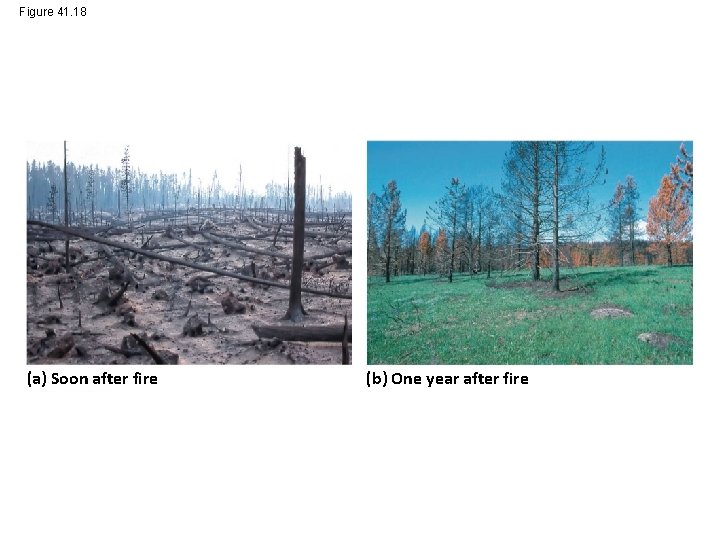
Figure 41. 18 (a) Soon after fire (b) One year after fire
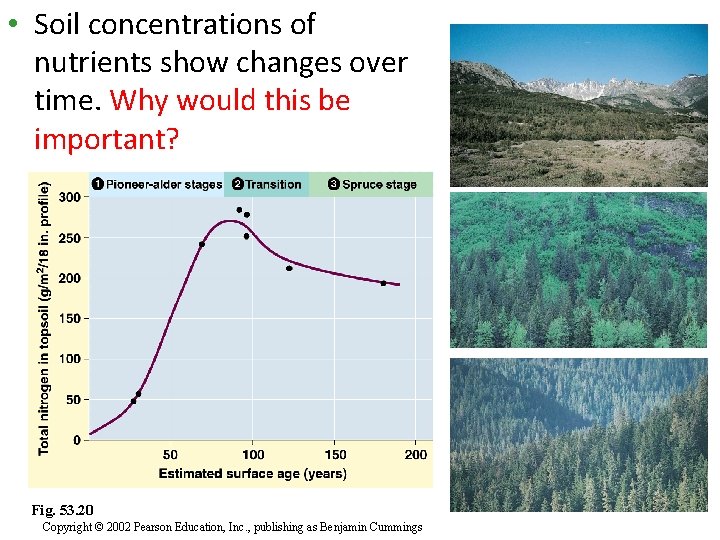
• Soil concentrations of nutrients show changes over time. Why would this be important? Fig. 53. 20 Copyright © 2002 Pearson Education, Inc. , publishing as Benjamin Cummings
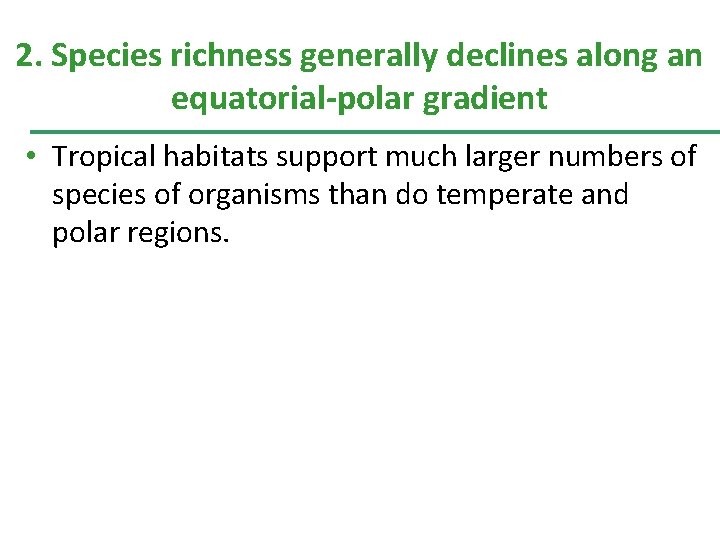
2. Species richness generally declines along an equatorial-polar gradient • Tropical habitats support much larger numbers of species of organisms than do temperate and polar regions.
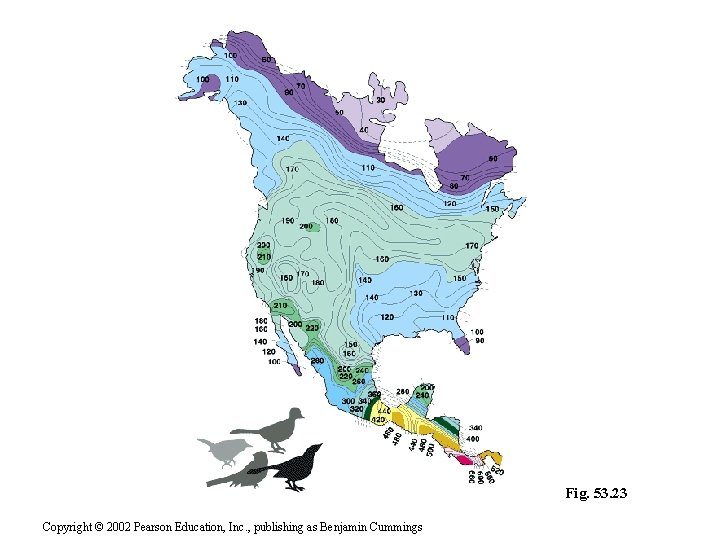
Fig. 53. 23 Copyright © 2002 Pearson Education, Inc. , publishing as Benjamin Cummings
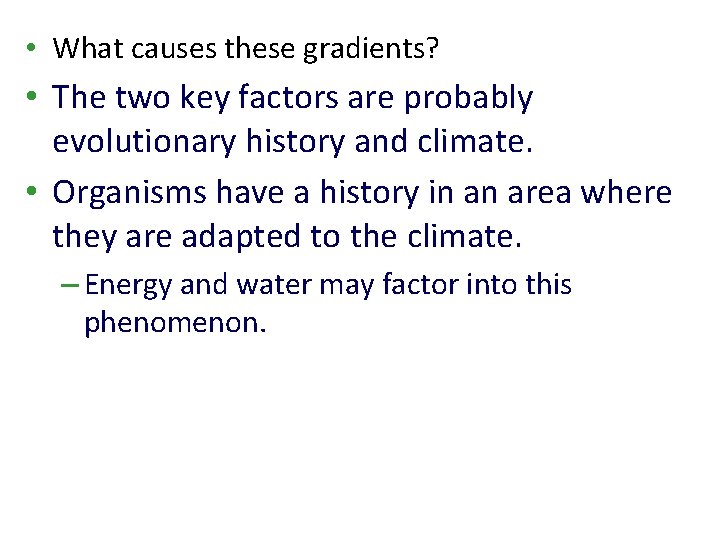
• What causes these gradients? • The two key factors are probably evolutionary history and climate. • Organisms have a history in an area where they are adapted to the climate. – Energy and water may factor into this phenomenon.
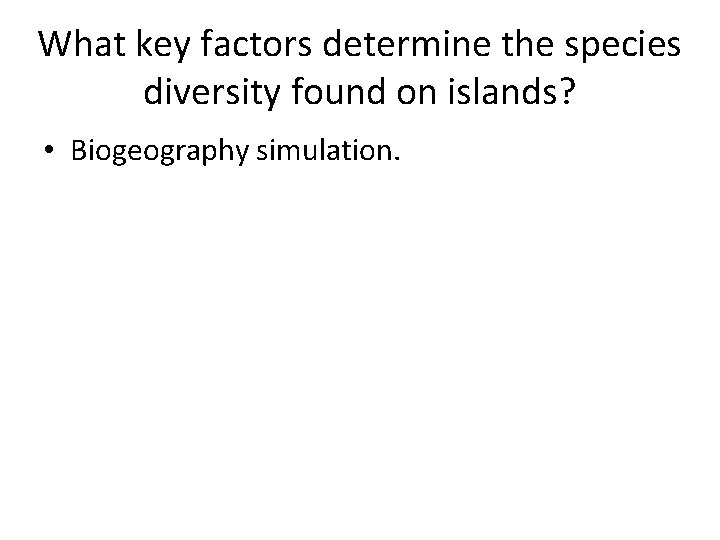
What key factors determine the species diversity found on islands? • Biogeography simulation.
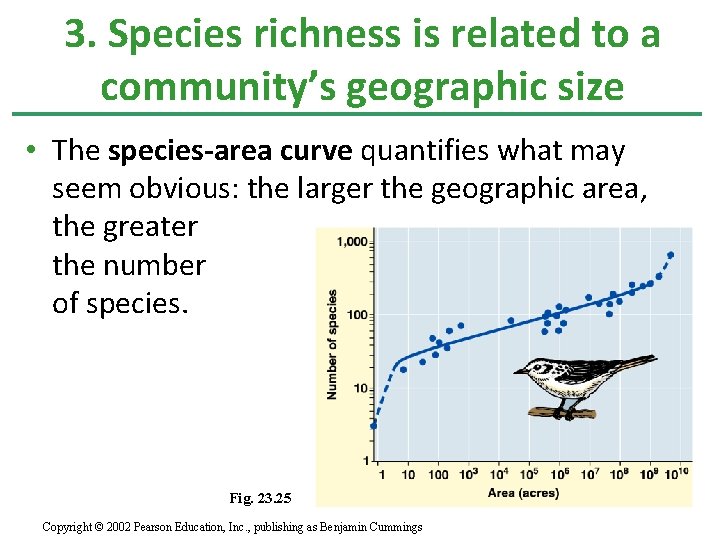
3. Species richness is related to a community’s geographic size • The species-area curve quantifies what may seem obvious: the larger the geographic area, the greater the number of species. Fig. 23. 25 Copyright © 2002 Pearson Education, Inc. , publishing as Benjamin Cummings
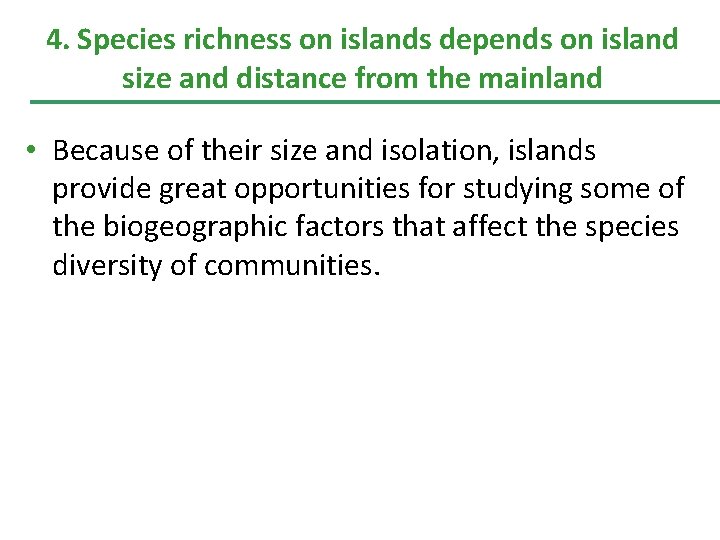
4. Species richness on islands depends on island size and distance from the mainland • Because of their size and isolation, islands provide great opportunities for studying some of the biogeographic factors that affect the species diversity of communities.
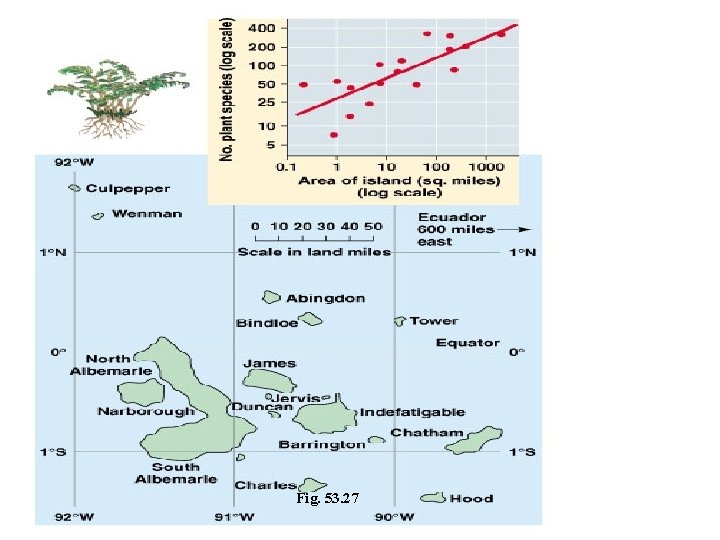
Fig. 53. 27
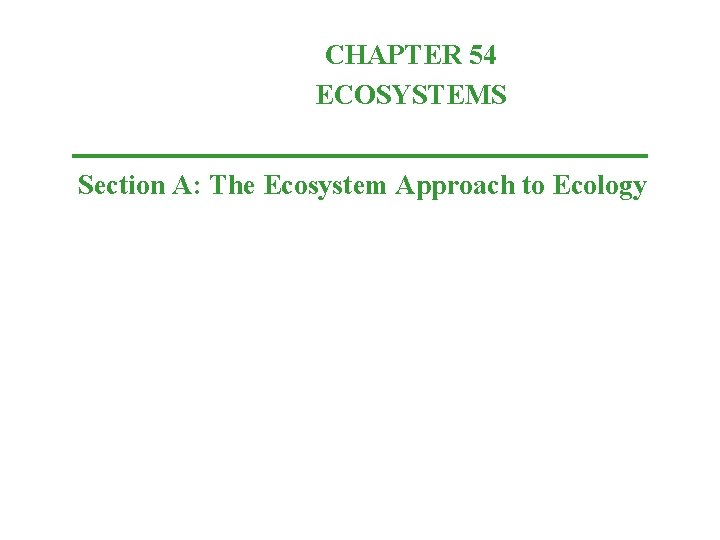
CHAPTER 54 ECOSYSTEMS Section A: The Ecosystem Approach to Ecology
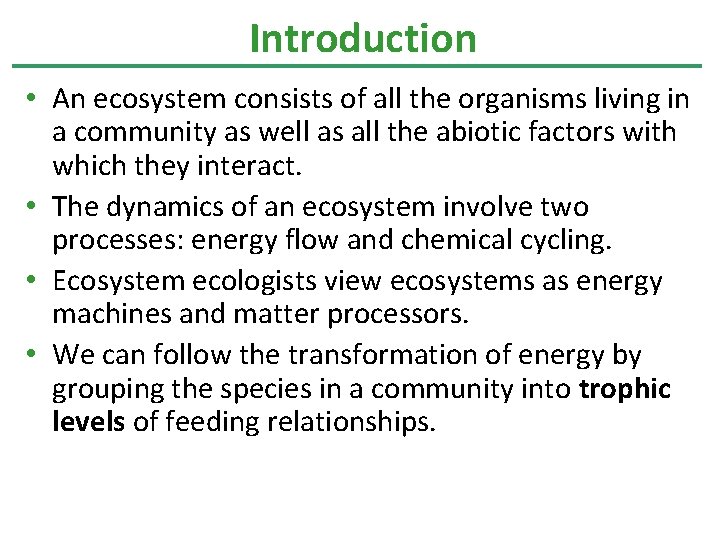
Introduction • An ecosystem consists of all the organisms living in a community as well as all the abiotic factors with which they interact. • The dynamics of an ecosystem involve two processes: energy flow and chemical cycling. • Ecosystem ecologists view ecosystems as energy machines and matter processors. • We can follow the transformation of energy by grouping the species in a community into trophic levels of feeding relationships.
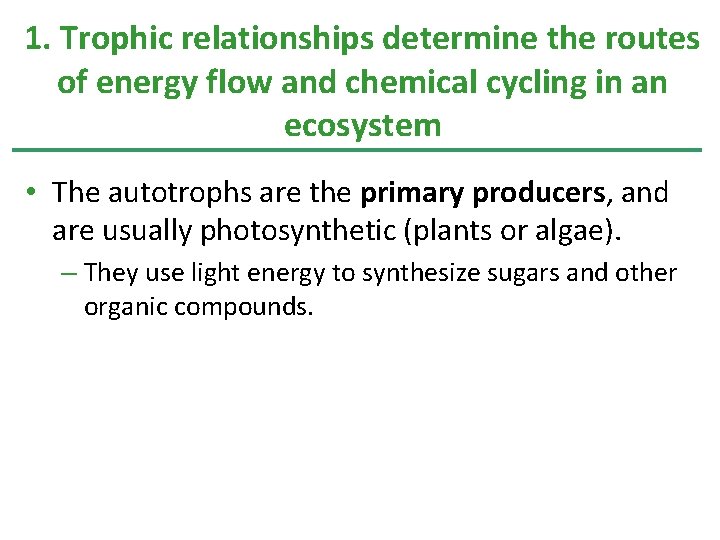
1. Trophic relationships determine the routes of energy flow and chemical cycling in an ecosystem • The autotrophs are the primary producers, and are usually photosynthetic (plants or algae). – They use light energy to synthesize sugars and other organic compounds.
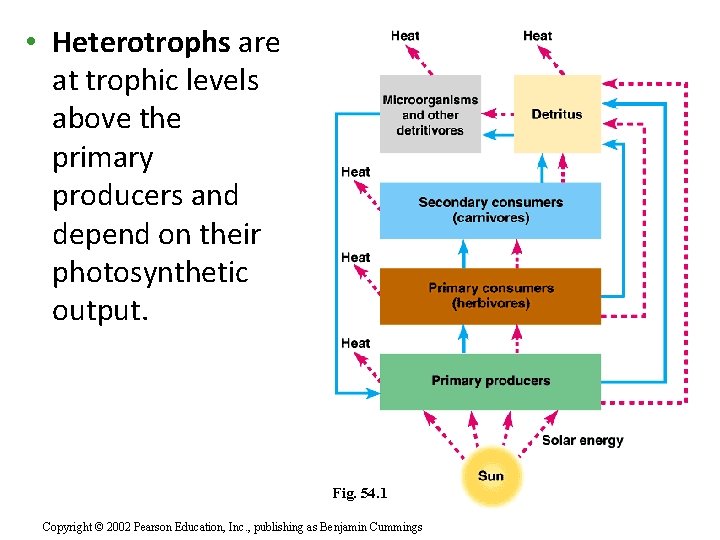
• Heterotrophs are at trophic levels above the primary producers and depend on their photosynthetic output. Fig. 54. 1 Copyright © 2002 Pearson Education, Inc. , publishing as Benjamin Cummings
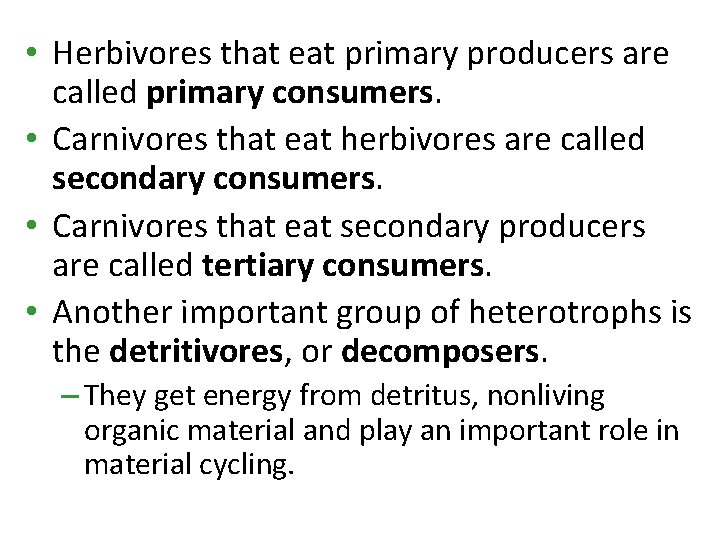
• Herbivores that eat primary producers are called primary consumers. • Carnivores that eat herbivores are called secondary consumers. • Carnivores that eat secondary producers are called tertiary consumers. • Another important group of heterotrophs is the detritivores, or decomposers. – They get energy from detritus, nonliving organic material and play an important role in material cycling.
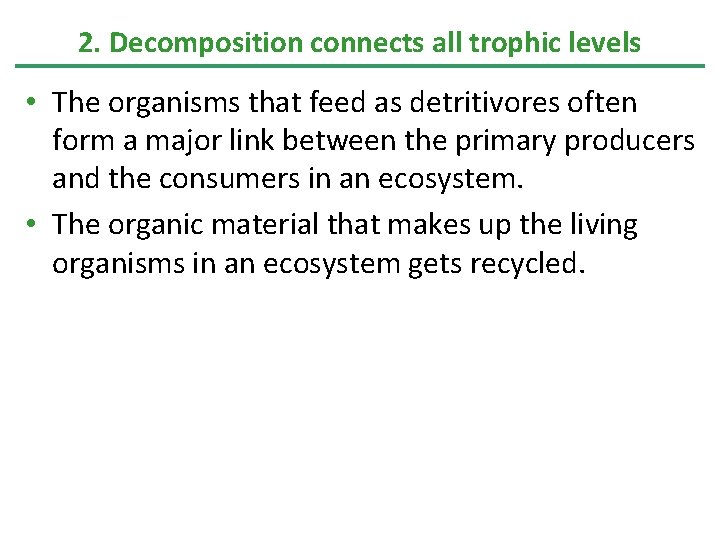
2. Decomposition connects all trophic levels • The organisms that feed as detritivores often form a major link between the primary producers and the consumers in an ecosystem. • The organic material that makes up the living organisms in an ecosystem gets recycled.
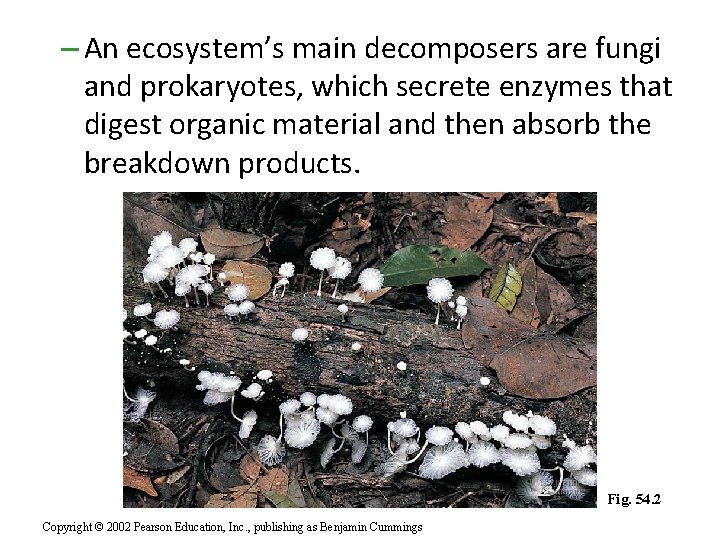
– An ecosystem’s main decomposers are fungi and prokaryotes, which secrete enzymes that digest organic material and then absorb the breakdown products. Fig. 54. 2 Copyright © 2002 Pearson Education, Inc. , publishing as Benjamin Cummings
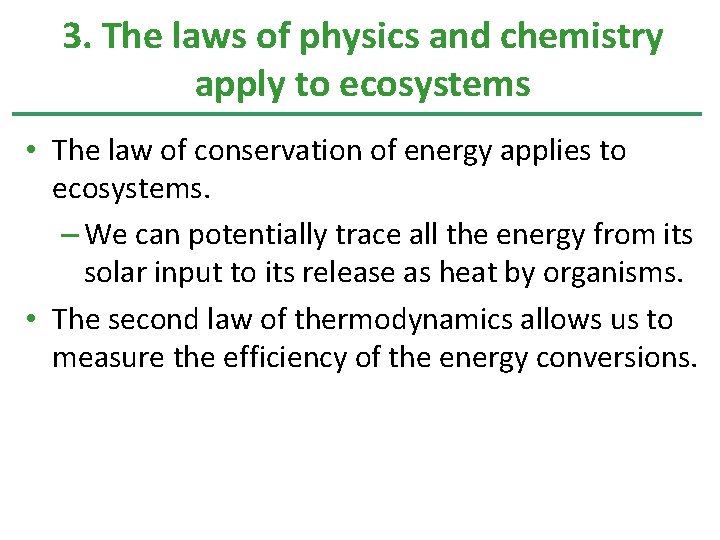
3. The laws of physics and chemistry apply to ecosystems • The law of conservation of energy applies to ecosystems. – We can potentially trace all the energy from its solar input to its release as heat by organisms. • The second law of thermodynamics allows us to measure the efficiency of the energy conversions.
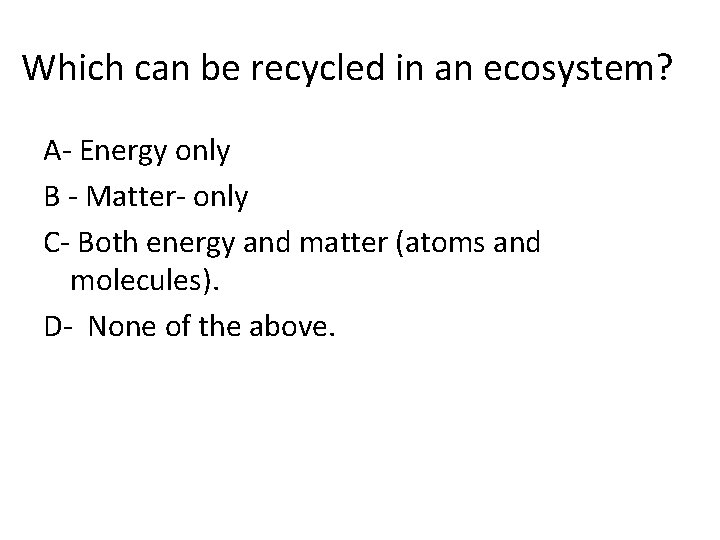
Which can be recycled in an ecosystem? A- Energy only B - Matter- only C- Both energy and matter (atoms and molecules). D- None of the above.
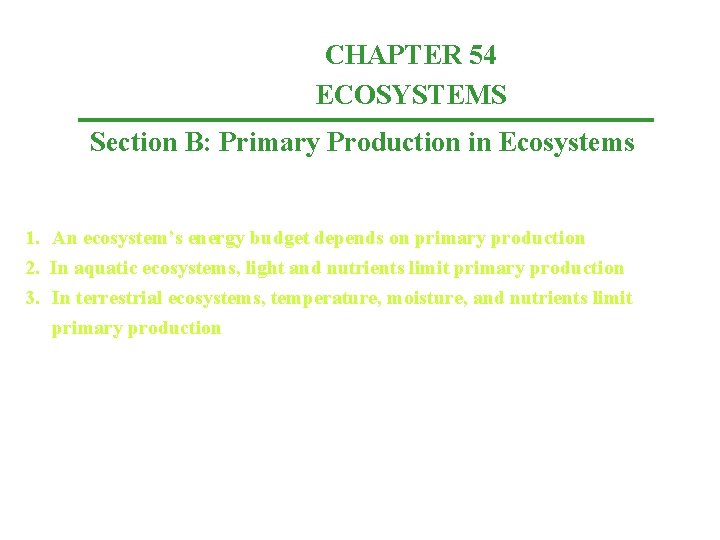
CHAPTER 54 ECOSYSTEMS Section B: Primary Production in Ecosystems 1. An ecosystem’s energy budget depends on primary production 2. In aquatic ecosystems, light and nutrients limit primary production 3. In terrestrial ecosystems, temperature, moisture, and nutrients limit primary production
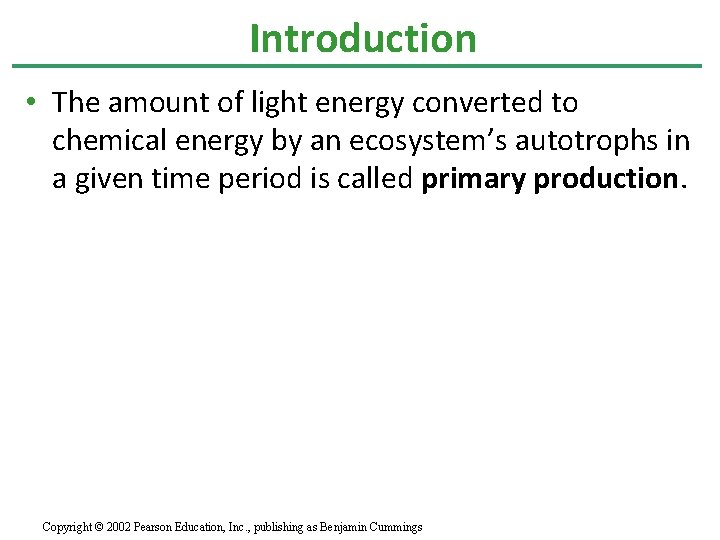
Introduction • The amount of light energy converted to chemical energy by an ecosystem’s autotrophs in a given time period is called primary production. Copyright © 2002 Pearson Education, Inc. , publishing as Benjamin Cummings
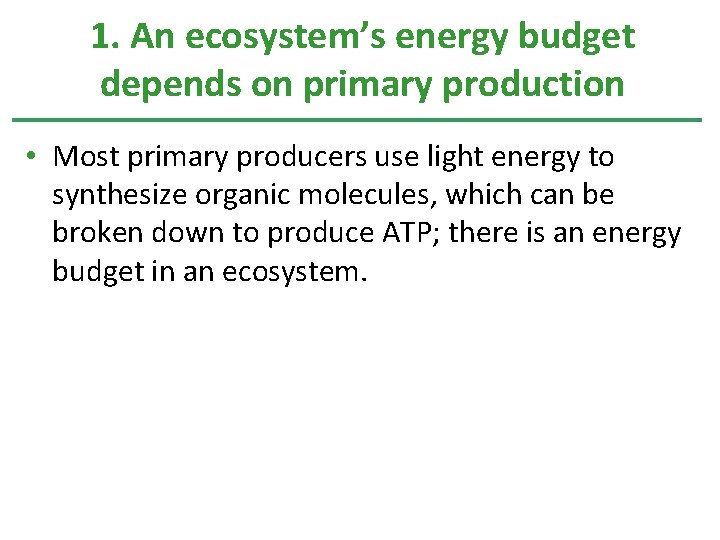
1. An ecosystem’s energy budget depends on primary production • Most primary producers use light energy to synthesize organic molecules, which can be broken down to produce ATP; there is an energy budget in an ecosystem.
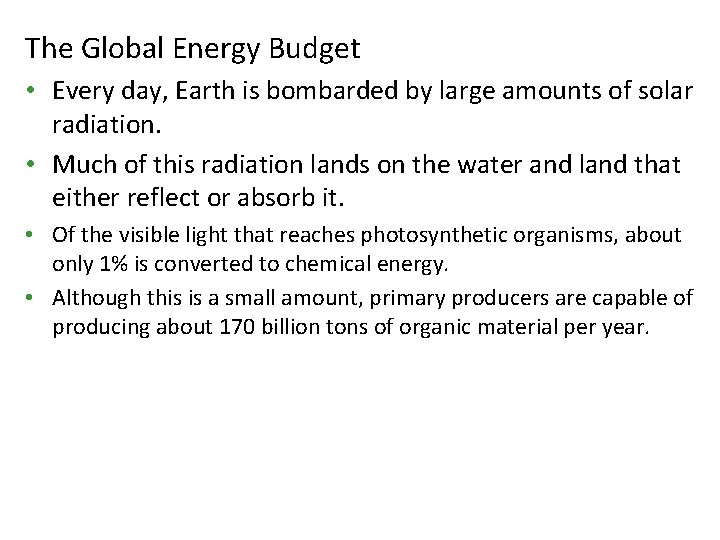
The Global Energy Budget • Every day, Earth is bombarded by large amounts of solar radiation. • Much of this radiation lands on the water and land that either reflect or absorb it. • Of the visible light that reaches photosynthetic organisms, about only 1% is converted to chemical energy. • Although this is a small amount, primary producers are capable of producing about 170 billion tons of organic material per year.
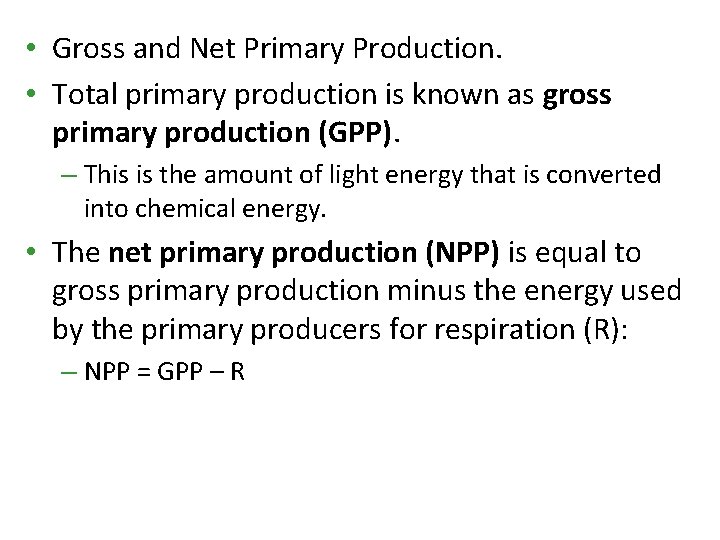
• Gross and Net Primary Production. • Total primary production is known as gross primary production (GPP). – This is the amount of light energy that is converted into chemical energy. • The net primary production (NPP) is equal to gross primary production minus the energy used by the primary producers for respiration (R): – NPP = GPP – R
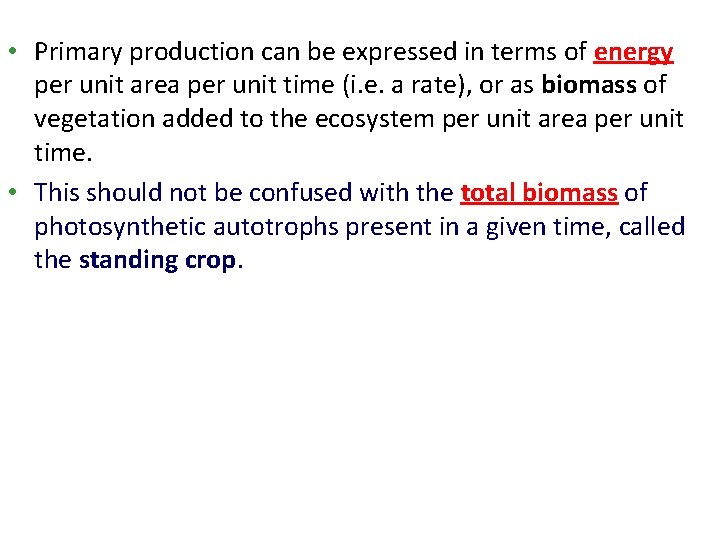
• Primary production can be expressed in terms of energy per unit area per unit time (i. e. a rate), or as biomass of vegetation added to the ecosystem per unit area per unit time. • This should not be confused with the total biomass of photosynthetic autotrophs present in a given time, called the standing crop.
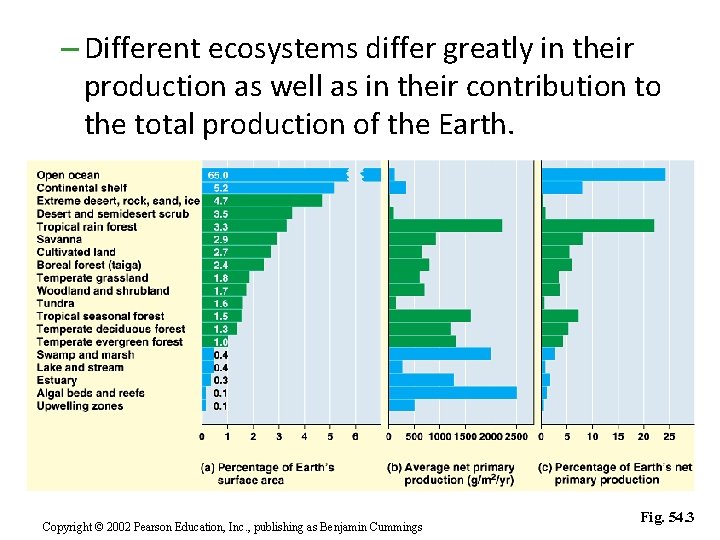
– Different ecosystems differ greatly in their production as well as in their contribution to the total production of the Earth. Copyright © 2002 Pearson Education, Inc. , publishing as Benjamin Cummings Fig. 54. 3
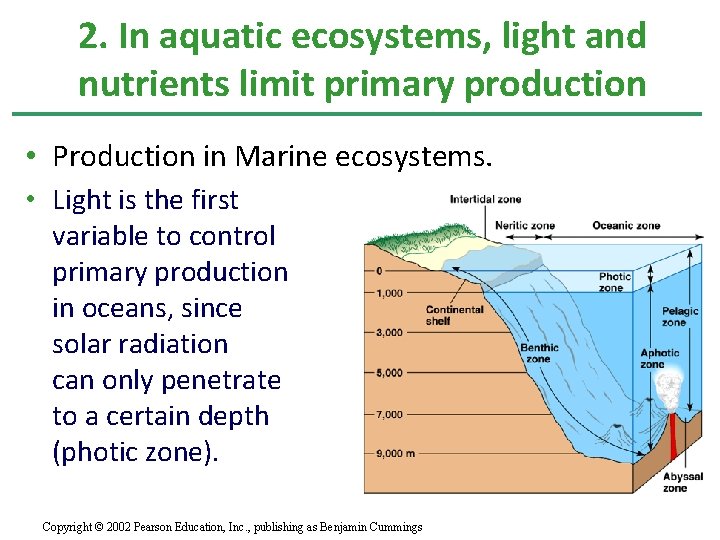
2. In aquatic ecosystems, light and nutrients limit primary production • Production in Marine ecosystems. • Light is the first variable to control primary production in oceans, since solar radiation can only penetrate to a certain depth (photic zone). Copyright © 2002 Pearson Education, Inc. , publishing as Benjamin Cummings
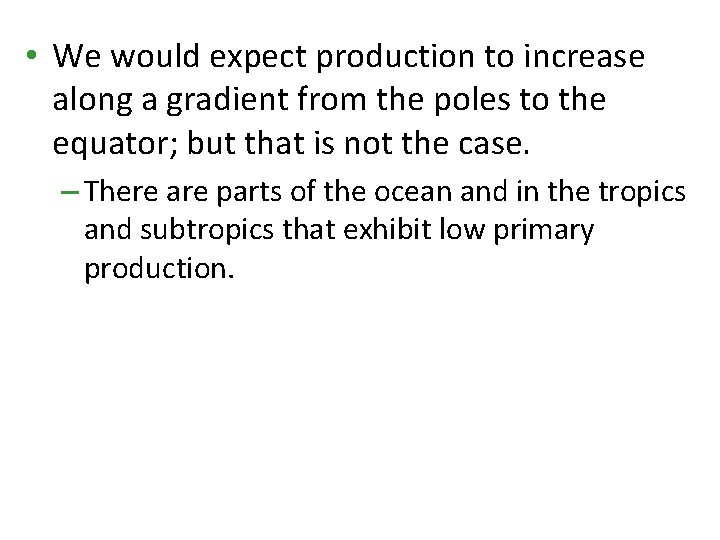
• We would expect production to increase along a gradient from the poles to the equator; but that is not the case. – There are parts of the ocean and in the tropics and subtropics that exhibit low primary production.
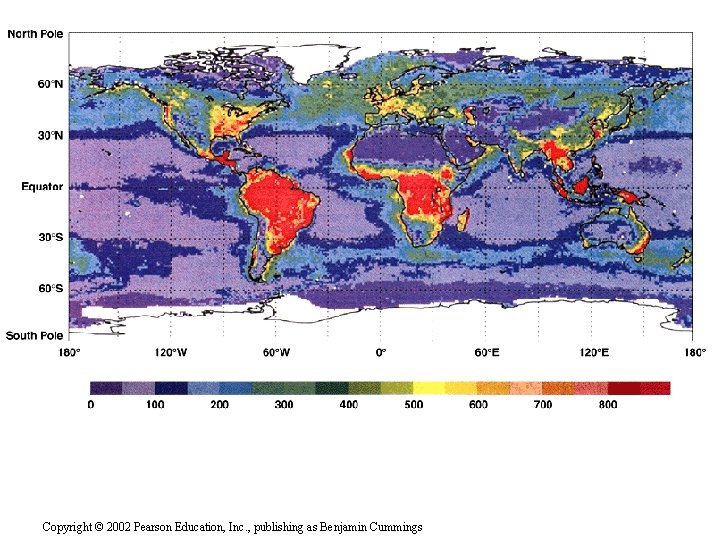
Copyright © 2002 Pearson Education, Inc. , publishing as Benjamin Cummings
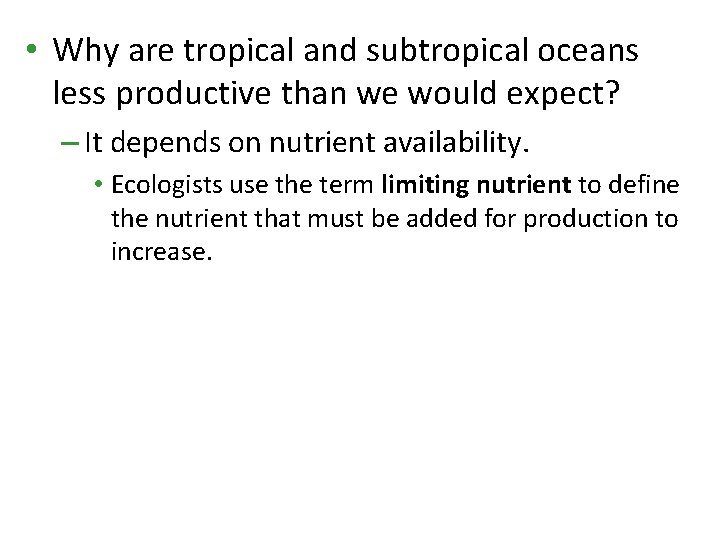
• Why are tropical and subtropical oceans less productive than we would expect? – It depends on nutrient availability. • Ecologists use the term limiting nutrient to define the nutrient that must be added for production to increase.
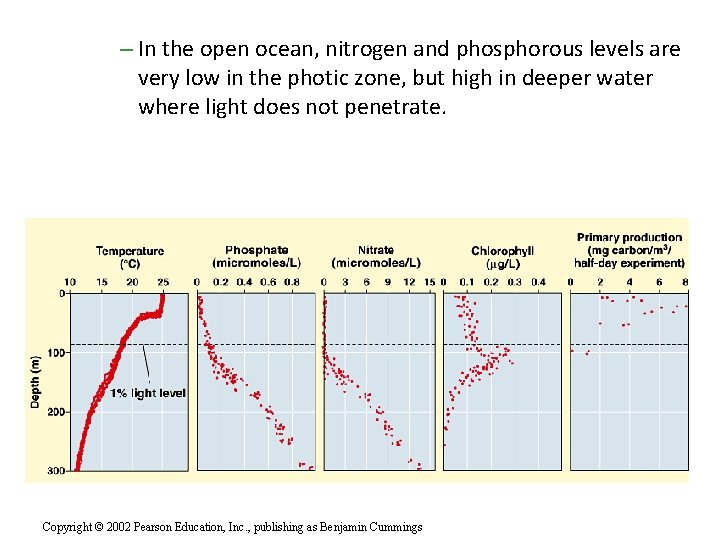
– In the open ocean, nitrogen and phosphorous levels are very low in the photic zone, but high in deeper water where light does not penetrate. Copyright © 2002 Pearson Education, Inc. , publishing as Benjamin Cummings
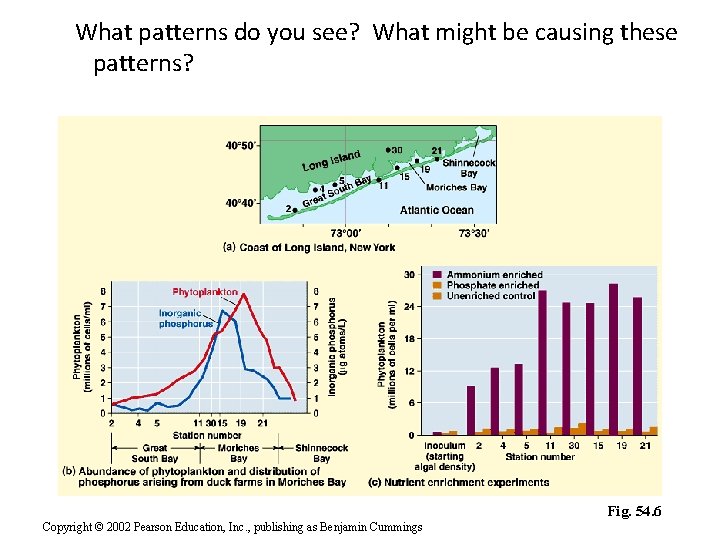
What patterns do you see? What might be causing these patterns? Fig. 54. 6 Copyright © 2002 Pearson Education, Inc. , publishing as Benjamin Cummings
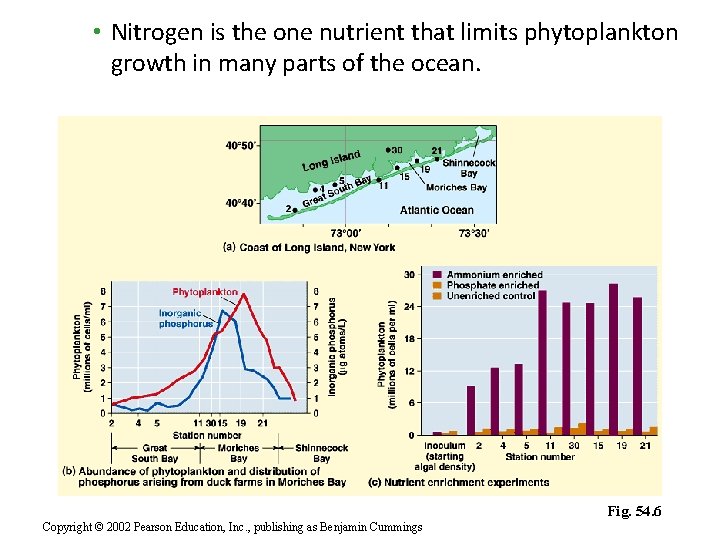
• Nitrogen is the one nutrient that limits phytoplankton growth in many parts of the ocean. Fig. 54. 6 Copyright © 2002 Pearson Education, Inc. , publishing as Benjamin Cummings
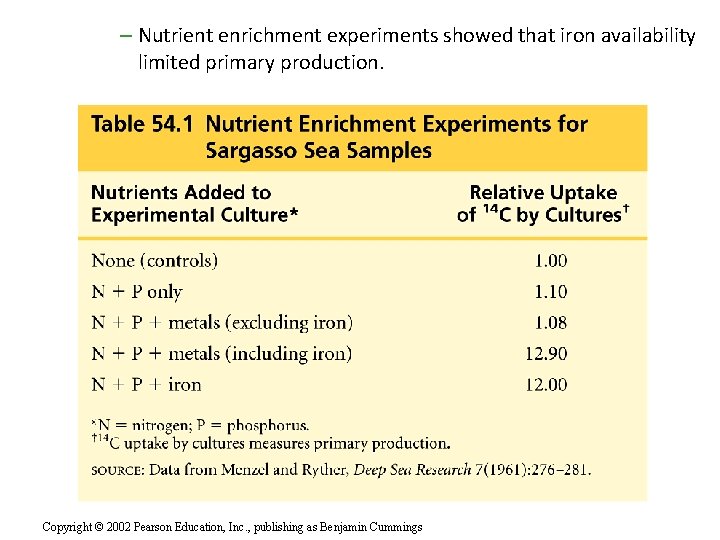
– Nutrient enrichment experiments showed that iron availability limited primary production. Copyright © 2002 Pearson Education, Inc. , publishing as Benjamin Cummings
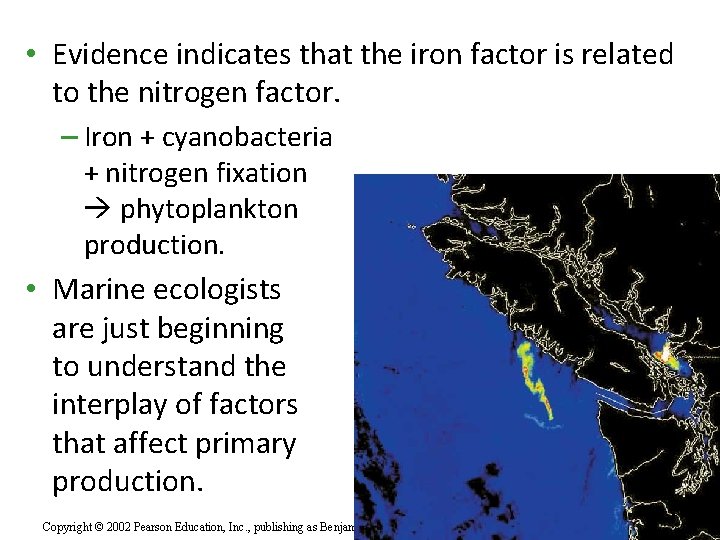
• Evidence indicates that the iron factor is related to the nitrogen factor. – Iron + cyanobacteria + nitrogen fixation phytoplankton production. • Marine ecologists are just beginning to understand the interplay of factors that affect primary production. Fig. 54. 7 Copyright © 2002 Pearson Education, Inc. , publishing as Benjamin Cummings
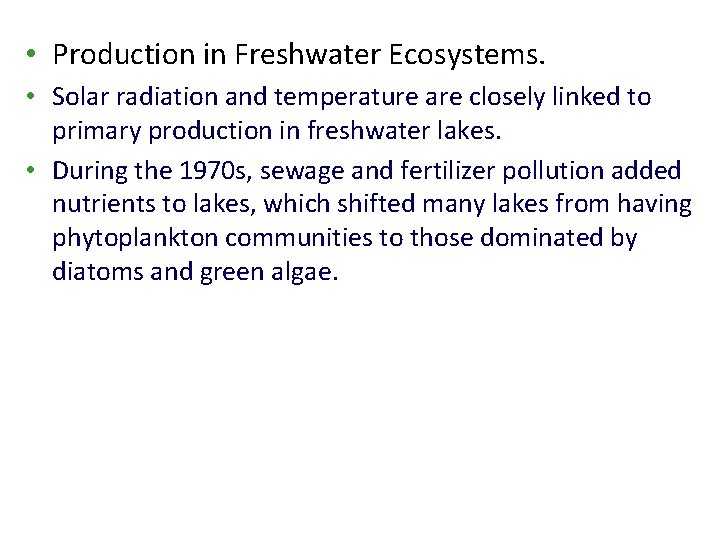
• Production in Freshwater Ecosystems. • Solar radiation and temperature are closely linked to primary production in freshwater lakes. • During the 1970 s, sewage and fertilizer pollution added nutrients to lakes, which shifted many lakes from having phytoplankton communities to those dominated by diatoms and green algae.
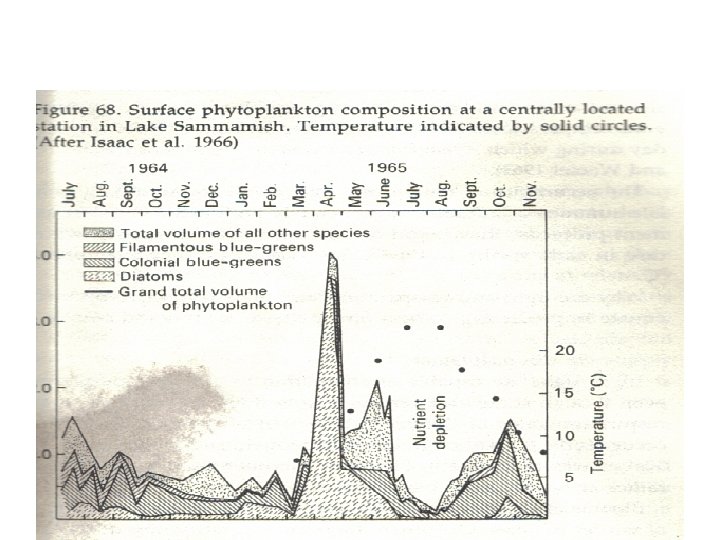
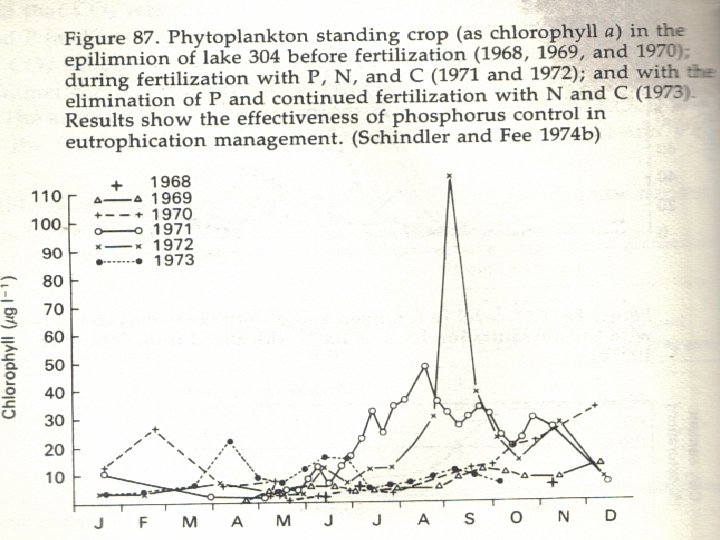
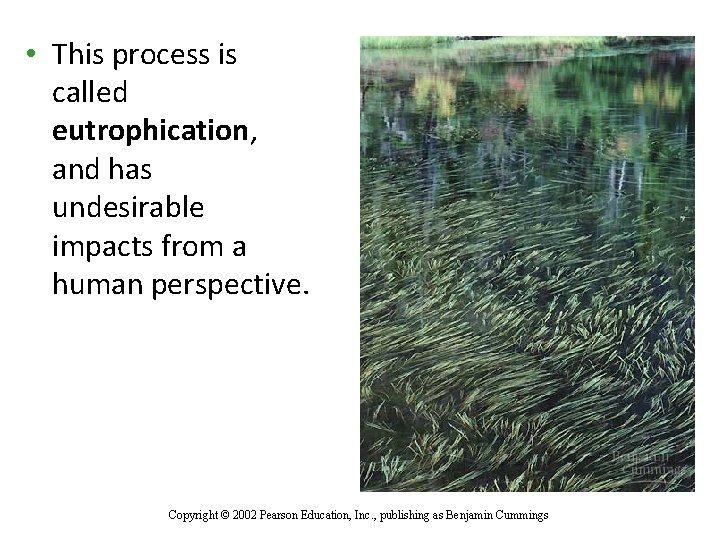
• This process is called eutrophication, and has undesirable impacts from a human perspective. Copyright © 2002 Pearson Education, Inc. , publishing as Benjamin Cummings
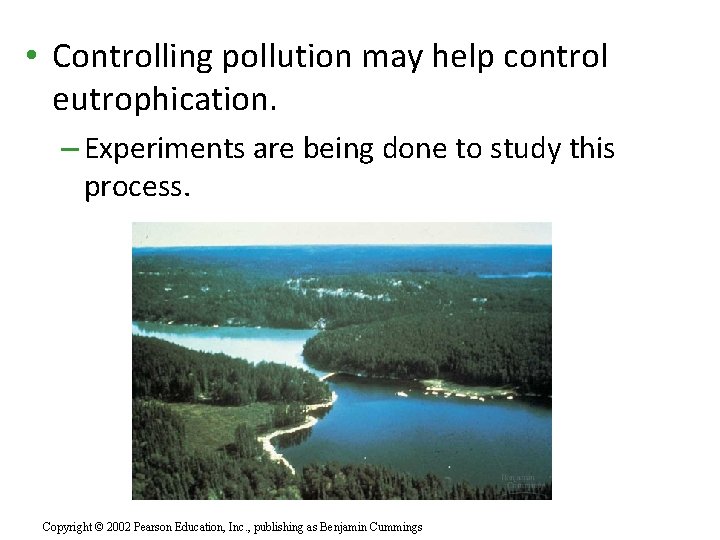
• Controlling pollution may help control eutrophication. – Experiments are being done to study this process. Copyright © 2002 Pearson Education, Inc. , publishing as Benjamin Cummings
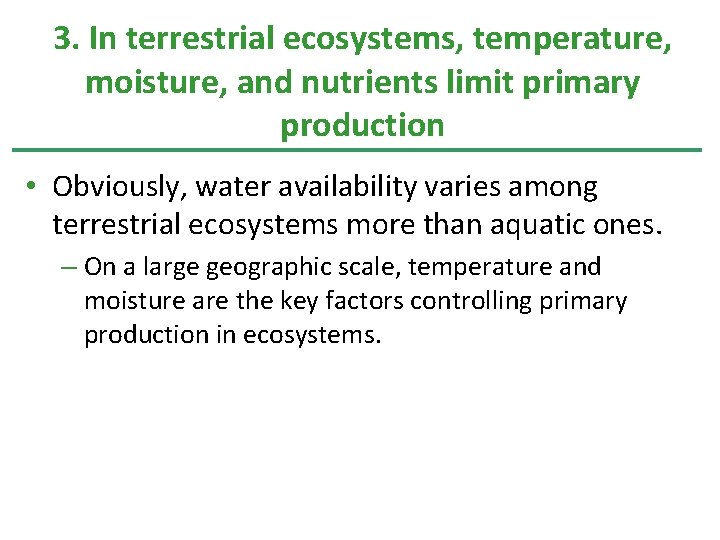
3. In terrestrial ecosystems, temperature, moisture, and nutrients limit primary production • Obviously, water availability varies among terrestrial ecosystems more than aquatic ones. – On a large geographic scale, temperature and moisture are the key factors controlling primary production in ecosystems.
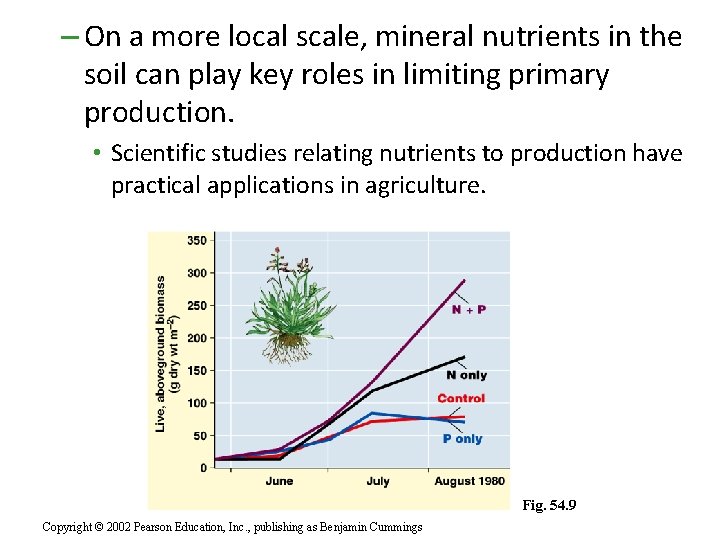
– On a more local scale, mineral nutrients in the soil can play key roles in limiting primary production. • Scientific studies relating nutrients to production have practical applications in agriculture. Fig. 54. 9 Copyright © 2002 Pearson Education, Inc. , publishing as Benjamin Cummings
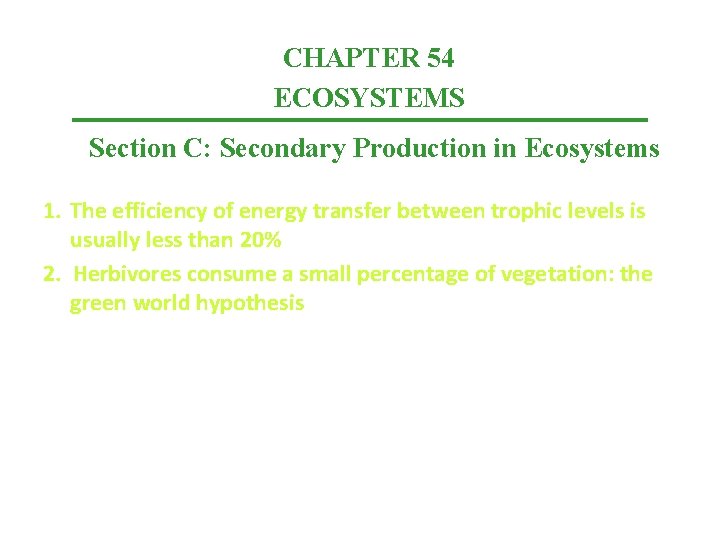
CHAPTER 54 ECOSYSTEMS Section C: Secondary Production in Ecosystems 1. The efficiency of energy transfer between trophic levels is usually less than 20% 2. Herbivores consume a small percentage of vegetation: the green world hypothesis
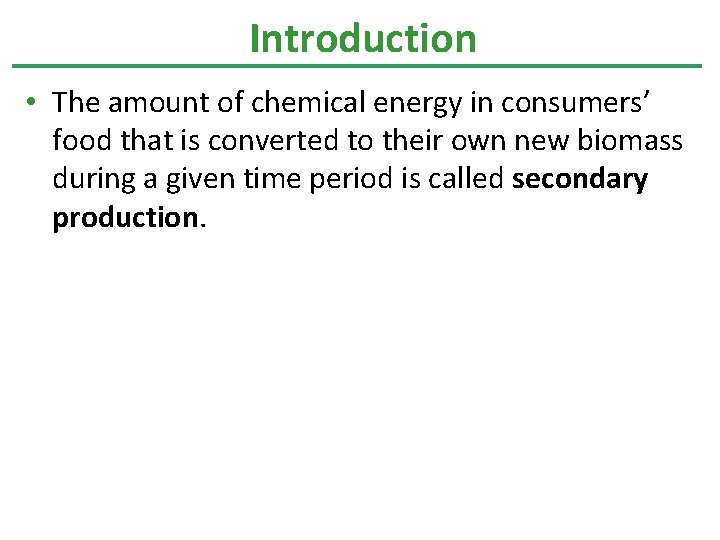
Introduction • The amount of chemical energy in consumers’ food that is converted to their own new biomass during a given time period is called secondary production.
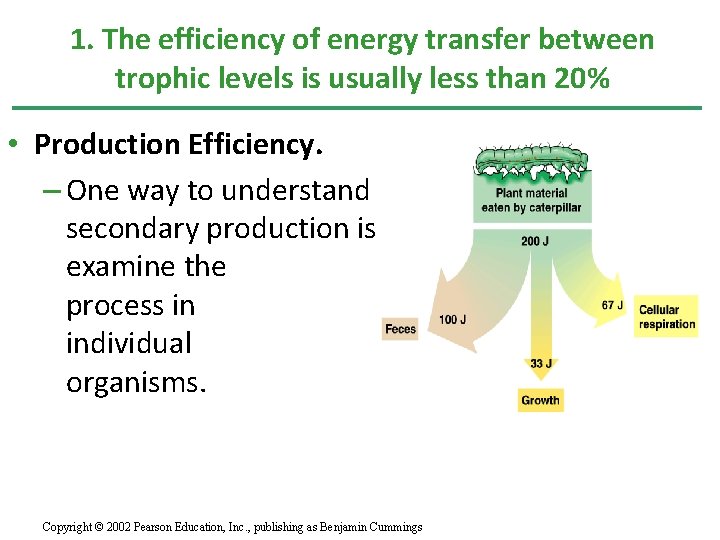
1. The efficiency of energy transfer between trophic levels is usually less than 20% • Production Efficiency. – One way to understand secondary production is to examine the process in individual organisms. Copyright © 2002 Pearson Education, Inc. , publishing as Benjamin Cummings
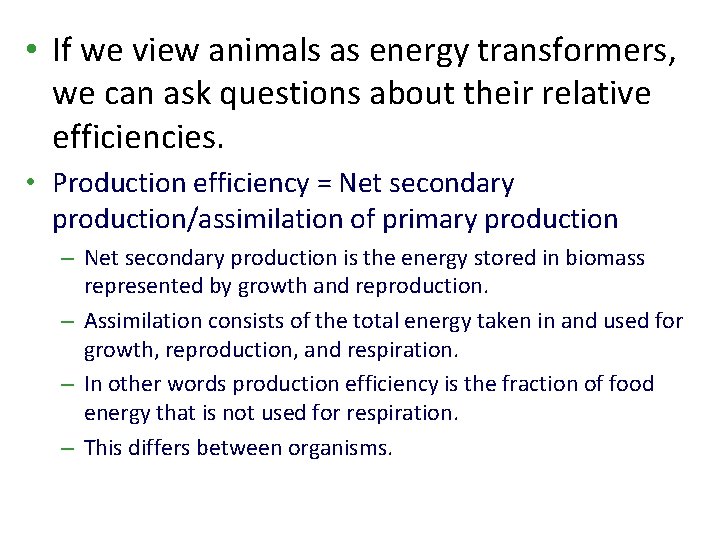
• If we view animals as energy transformers, we can ask questions about their relative efficiencies. • Production efficiency = Net secondary production/assimilation of primary production – Net secondary production is the energy stored in biomass represented by growth and reproduction. – Assimilation consists of the total energy taken in and used for growth, reproduction, and respiration. – In other words production efficiency is the fraction of food energy that is not used for respiration. – This differs between organisms.
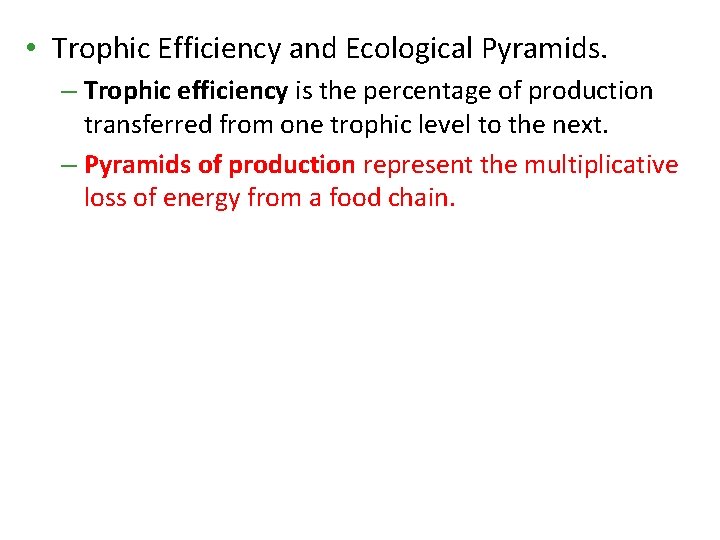
• Trophic Efficiency and Ecological Pyramids. – Trophic efficiency is the percentage of production transferred from one trophic level to the next. – Pyramids of production represent the multiplicative loss of energy from a food chain.
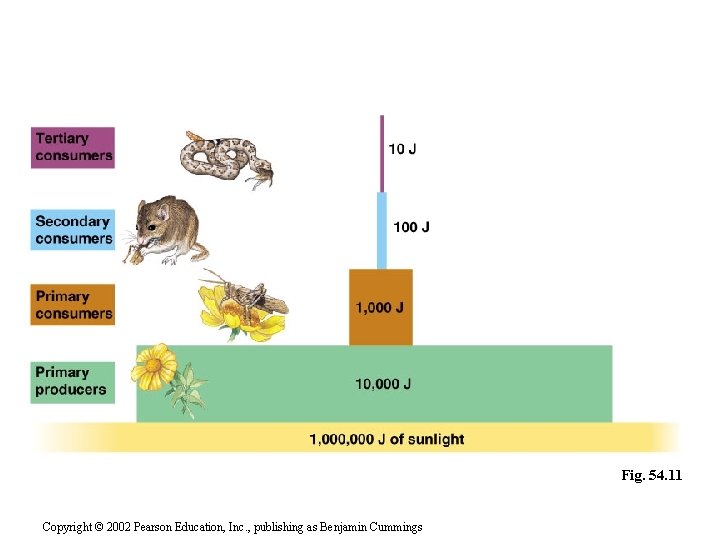
Fig. 54. 11 Copyright © 2002 Pearson Education, Inc. , publishing as Benjamin Cummings
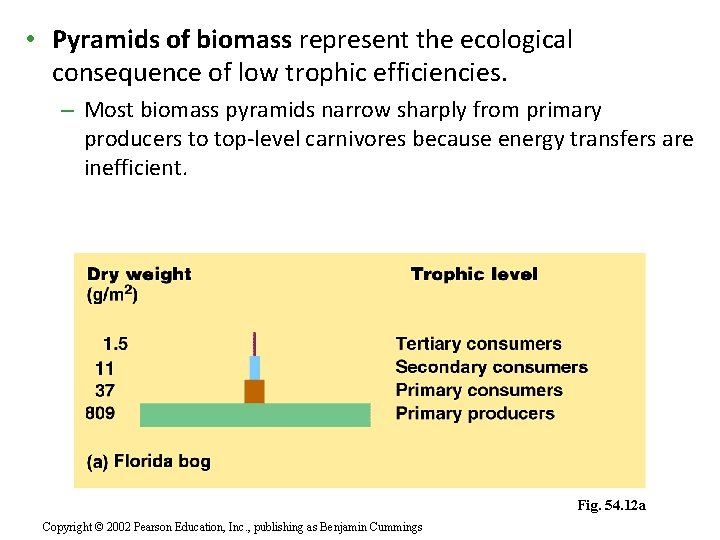
• Pyramids of biomass represent the ecological consequence of low trophic efficiencies. – Most biomass pyramids narrow sharply from primary producers to top-level carnivores because energy transfers are inefficient. Fig. 54. 12 a Copyright © 2002 Pearson Education, Inc. , publishing as Benjamin Cummings
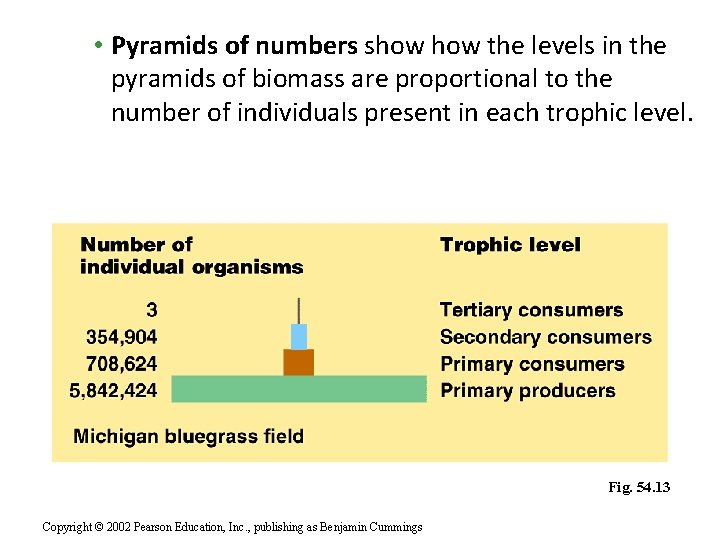
• Pyramids of numbers show the levels in the pyramids of biomass are proportional to the number of individuals present in each trophic level. Fig. 54. 13 Copyright © 2002 Pearson Education, Inc. , publishing as Benjamin Cummings
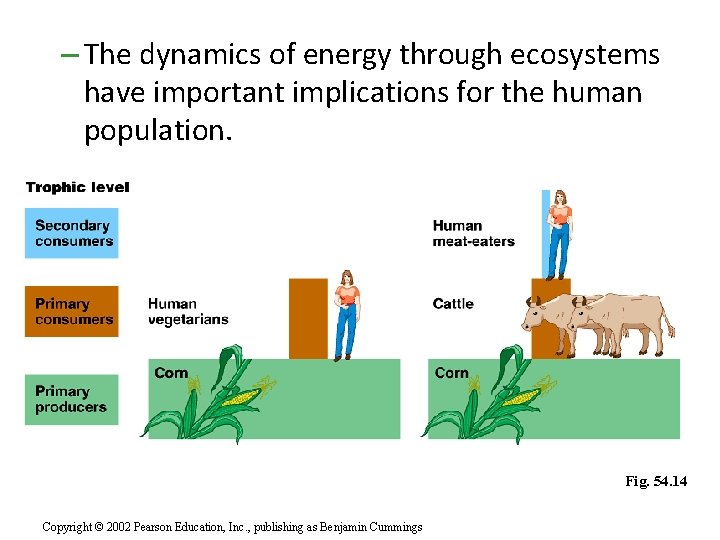
– The dynamics of energy through ecosystems have important implications for the human population. Fig. 54. 14 Copyright © 2002 Pearson Education, Inc. , publishing as Benjamin Cummings
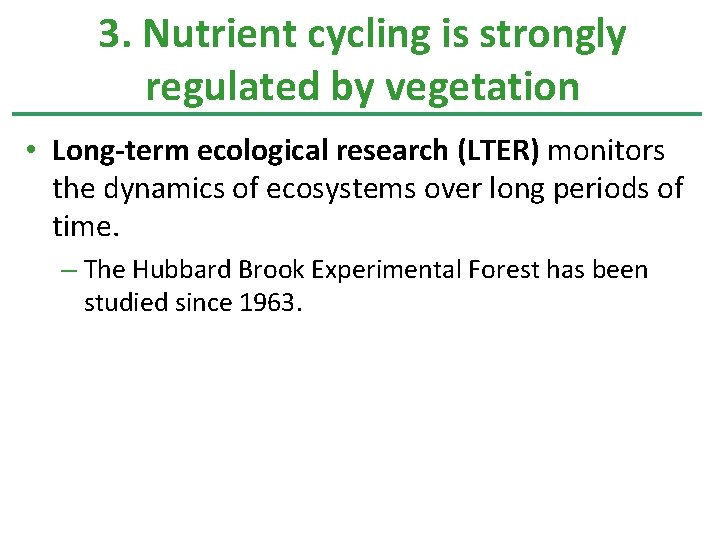
3. Nutrient cycling is strongly regulated by vegetation • Long-term ecological research (LTER) monitors the dynamics of ecosystems over long periods of time. – The Hubbard Brook Experimental Forest has been studied since 1963.
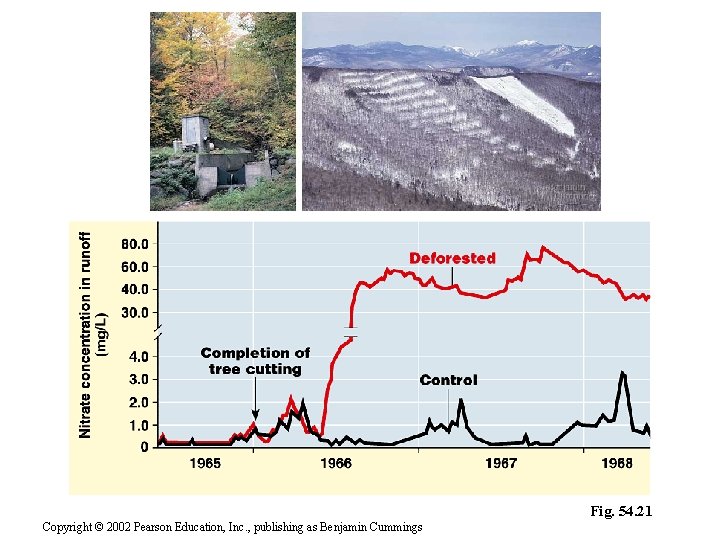
Fig. 54. 21 Copyright © 2002 Pearson Education, Inc. , publishing as Benjamin Cummings
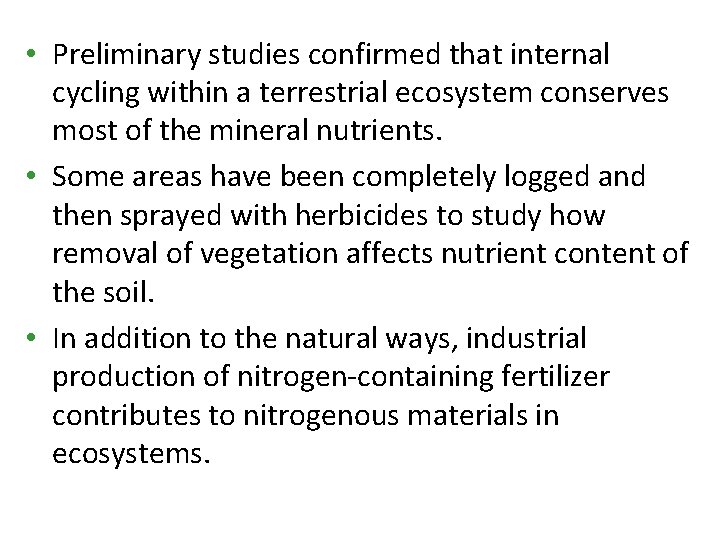
• Preliminary studies confirmed that internal cycling within a terrestrial ecosystem conserves most of the mineral nutrients. • Some areas have been completely logged and then sprayed with herbicides to study how removal of vegetation affects nutrient content of the soil. • In addition to the natural ways, industrial production of nitrogen-containing fertilizer contributes to nitrogenous materials in ecosystems.
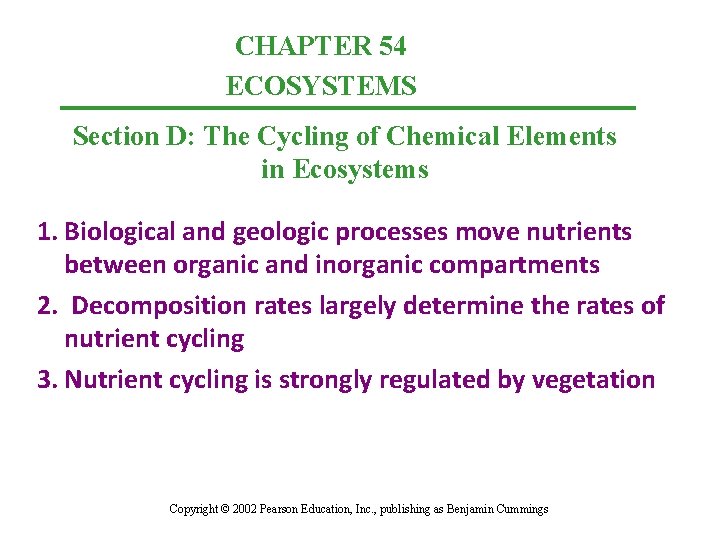
CHAPTER 54 ECOSYSTEMS Section D: The Cycling of Chemical Elements in Ecosystems 1. Biological and geologic processes move nutrients between organic and inorganic compartments 2. Decomposition rates largely determine the rates of nutrient cycling 3. Nutrient cycling is strongly regulated by vegetation Copyright © 2002 Pearson Education, Inc. , publishing as Benjamin Cummings
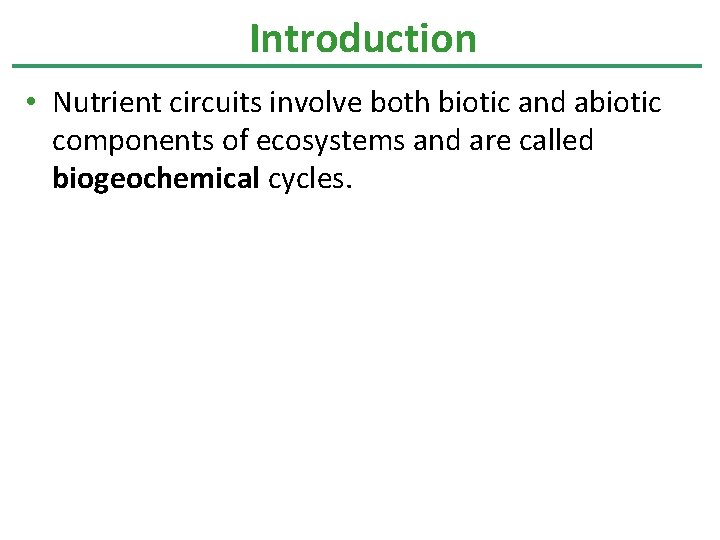
Introduction • Nutrient circuits involve both biotic and abiotic components of ecosystems and are called biogeochemical cycles.
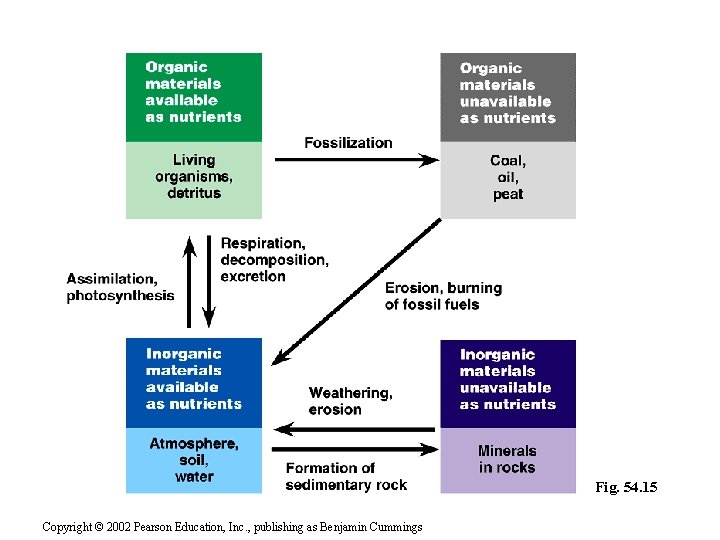
Fig. 54. 15 Copyright © 2002 Pearson Education, Inc. , publishing as Benjamin Cummings
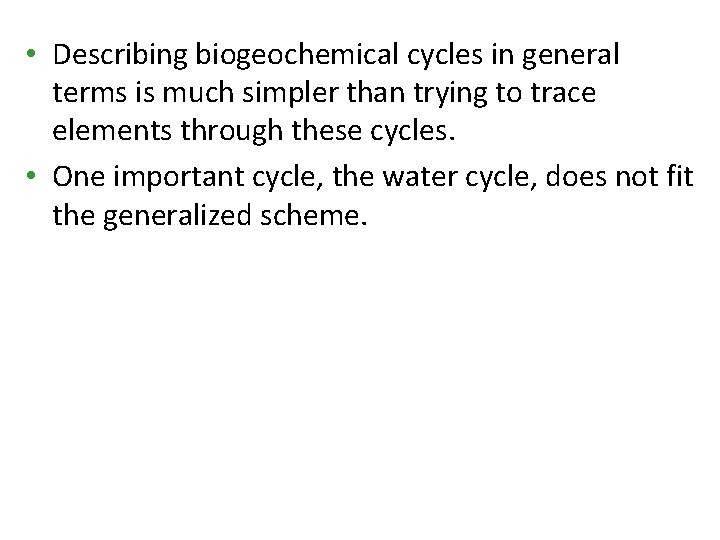
• Describing biogeochemical cycles in general terms is much simpler than trying to trace elements through these cycles. • One important cycle, the water cycle, does not fit the generalized scheme.
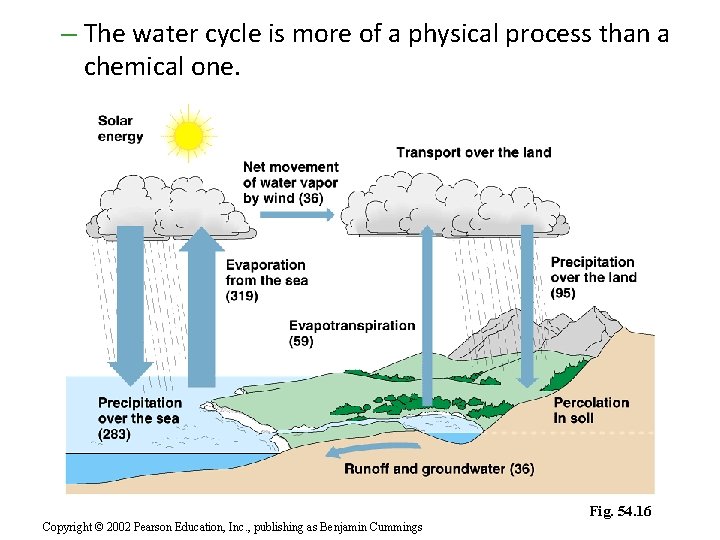
– The water cycle is more of a physical process than a chemical one. Fig. 54. 16 Copyright © 2002 Pearson Education, Inc. , publishing as Benjamin Cummings
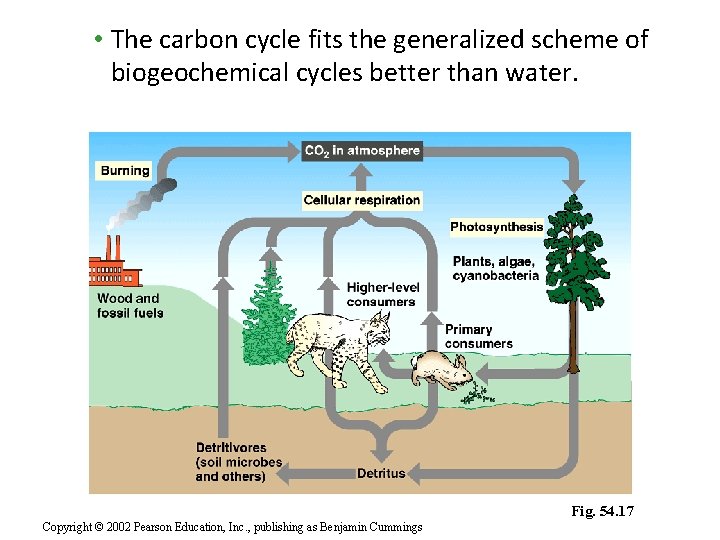
• The carbon cycle fits the generalized scheme of biogeochemical cycles better than water. Fig. 54. 17 Copyright © 2002 Pearson Education, Inc. , publishing as Benjamin Cummings
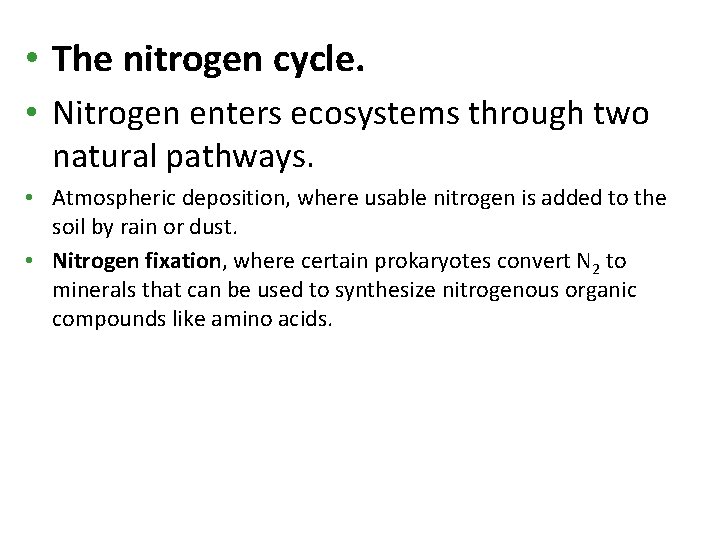
• The nitrogen cycle. • Nitrogen enters ecosystems through two natural pathways. • Atmospheric deposition, where usable nitrogen is added to the soil by rain or dust. • Nitrogen fixation, where certain prokaryotes convert N 2 to minerals that can be used to synthesize nitrogenous organic compounds like amino acids.
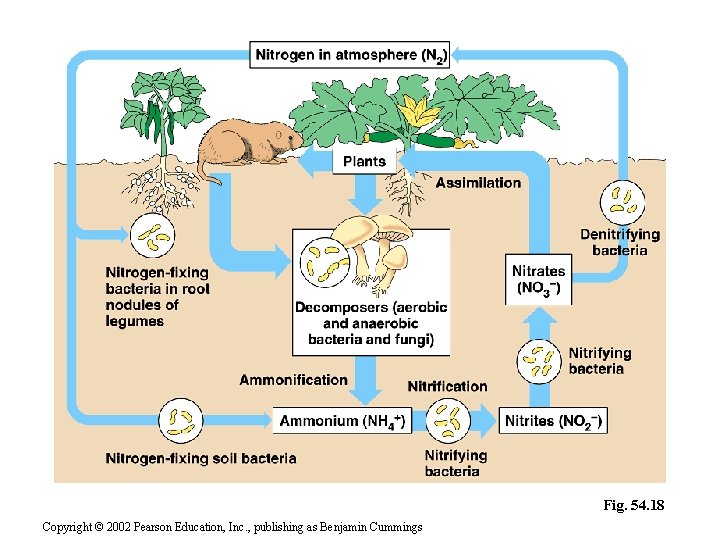
Fig. 54. 18 Copyright © 2002 Pearson Education, Inc. , publishing as Benjamin Cummings
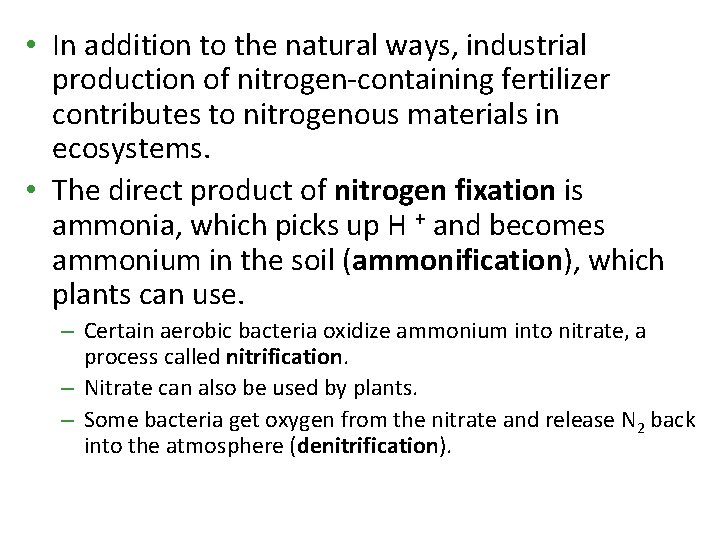
• In addition to the natural ways, industrial production of nitrogen-containing fertilizer contributes to nitrogenous materials in ecosystems. • The direct product of nitrogen fixation is ammonia, which picks up H + and becomes ammonium in the soil (ammonification), which plants can use. – Certain aerobic bacteria oxidize ammonium into nitrate, a process called nitrification. – Nitrate can also be used by plants. – Some bacteria get oxygen from the nitrate and release N 2 back into the atmosphere (denitrification).
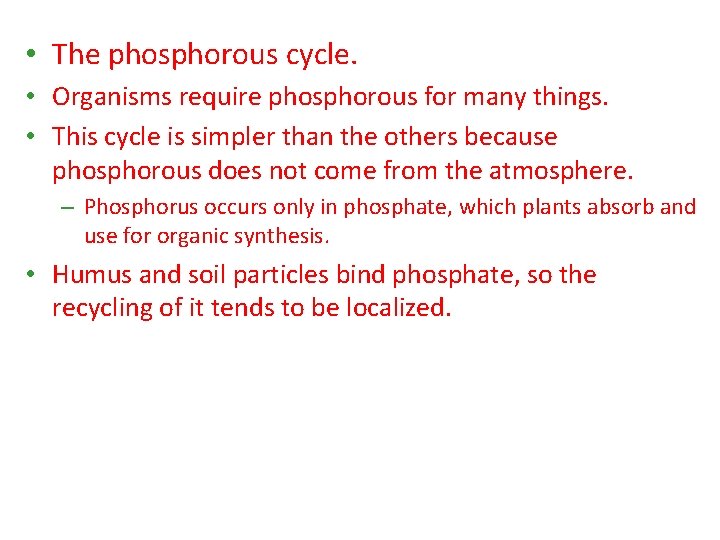
• The phosphorous cycle. • Organisms require phosphorous for many things. • This cycle is simpler than the others because phosphorous does not come from the atmosphere. – Phosphorus occurs only in phosphate, which plants absorb and use for organic synthesis. • Humus and soil particles bind phosphate, so the recycling of it tends to be localized.
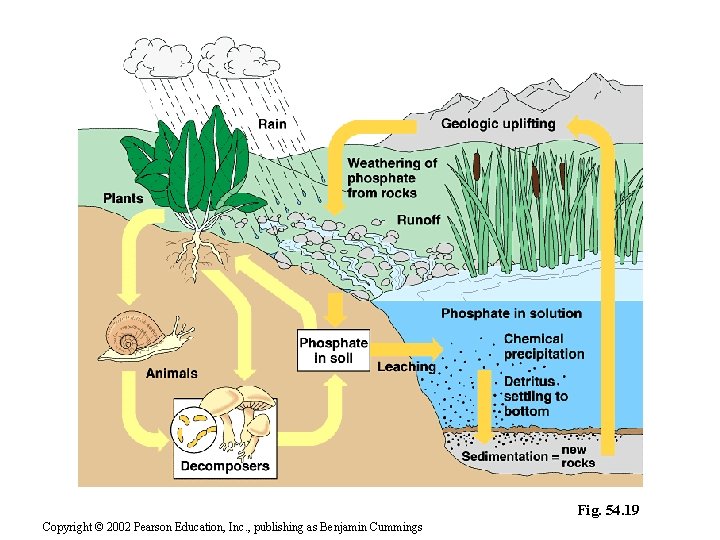
Fig. 54. 19 Copyright © 2002 Pearson Education, Inc. , publishing as Benjamin Cummings
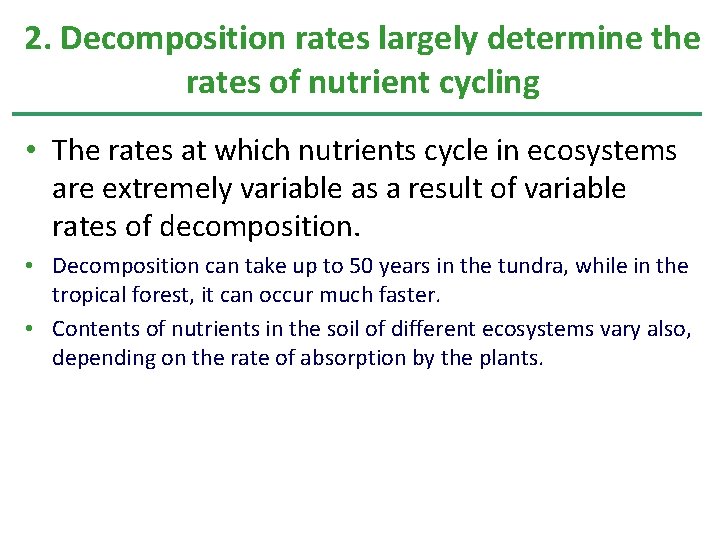
2. Decomposition rates largely determine the rates of nutrient cycling • The rates at which nutrients cycle in ecosystems are extremely variable as a result of variable rates of decomposition. • Decomposition can take up to 50 years in the tundra, while in the tropical forest, it can occur much faster. • Contents of nutrients in the soil of different ecosystems vary also, depending on the rate of absorption by the plants.
Syntax directed definition and syntax directed translation
Evolution and community ecology guided notes
Difference between ecosystem and ecology
Difference between ecosystem and ecology
Ecology terms
Veterans directed home and community based care
Veterans directed home and community based care
Compare and contrast community and ecosystem
Lesson 9: biosphere
An ecosystem consists of
Ecosystem ecology
Ecosystem ecology
Principles of ecology 2 flow of energy in an ecosystem
Ecosystem ecology
Ecosystem ecology
Ecology ecosystem
Ecosystem ecology
Ubc student directed seminars
Chapter 5 evolution and community ecology
Ecosystems interactions
Population community ecosystem
Biosphere
Population community ecosystem biome biosphere
Population vs community ecology
Organism population community ecosystem biosphere
Ecosystem dynamics definition
Whats is ecology
A student is studying the ecology of a playa lake
A student is studying the ecology of a playa lake
Is india near the equator
Community ecology
Chapter 3 section 1 community ecology answer key
Chapter 54 community ecology
Definition of community ecology
More diverse
The definition of community
Ecology examples
Community level ecology
Chapter 54 community ecology
Facteur g
How was your last weekend
What did they do last weekend
National student clearinghouse student tracker
Class maths student student1 class student string name
National student clearinghouse student tracker
Freckle.com.login
Teacher
Sls learning login
Do you belong to
Directed mutagenesis and protein engineering
Receiving and putaway
Is zero a directed number
Directed paraphrasing advantages and disadvantages
Indirect speech time change
Prepare to scale up in social mobilization
Directed line segment
Child directed speech definition
Syntax directed definition
Self directed search free
Sds holland food bv
Balthasar romeo and juliet
Compliant personality definition
Goal directed personas
Metamorphic grade
Properties of directed acyclic graph
While a lvn has a directed scope of practice
Mood descriptors in play
Weighted directed graph
Sociocultural perspective
Sglib1
Evaluation orders for sdd in compiler design
Dynamothermal
Directed designating
Direct motion specification
Affective play space
Positive and negative numbers definition
Partitioning a line segment
Partitioning a line segment formula
Is 0 a directed number
Directed number definition
Directed school laboratory sae
Self-directed play
Interview questions for self-directed learning
Power distance map
Loop graph
Force-directed algorithm
Working memory graphs
Directed number exercise
Oriented graph example
Sdr surveillance
Directed number definition
Diketahui suatu array segitiga memiliki 5 baris dan kolom
Gene shuffling
Directed line segment formula
Inner drive definition
Chapter 2 planning your career
Dr gan dunnington
Oligonucleotide directed mutagenesis
Power set
Self directed support options
Monitoring comprehension strategies
Site directed mutagenesis
Directed energy professional society
Rooute finder
Syntax directed definition
Dag directed acyclic graph
Force directed scheduling
Randoop automatic test
Directed physical exam
Market directed economy
Ecosystem living and nonliving things
Venn diagram of living and nonliving things
River ecosystem biotic and abiotic factors
Biotic and abiotic components of marine ecosystem
Ecosystem living and nonliving things
Collage of biotic and abiotic components