Atomic structure Nucleus This contains protons and neutrons
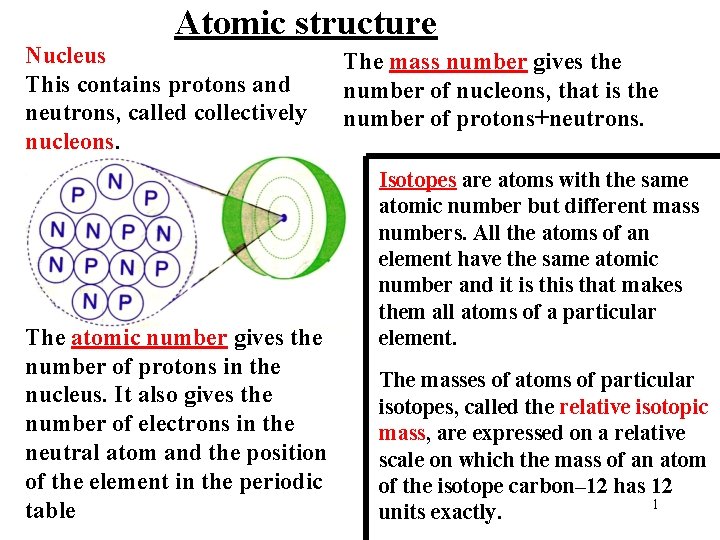
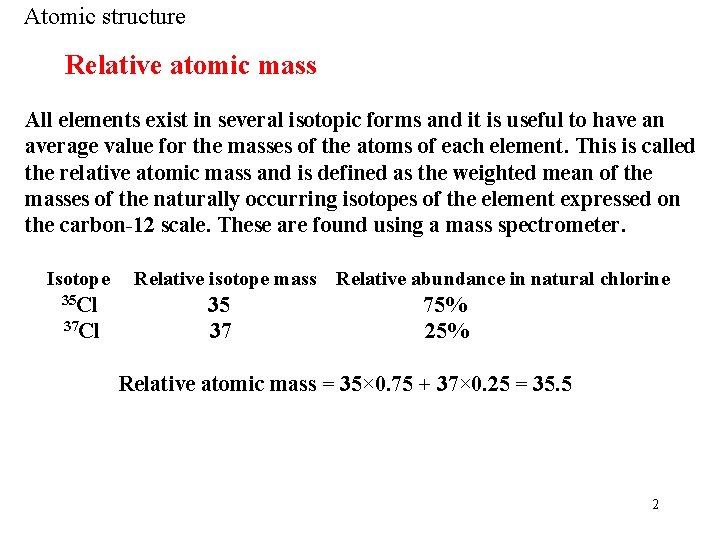
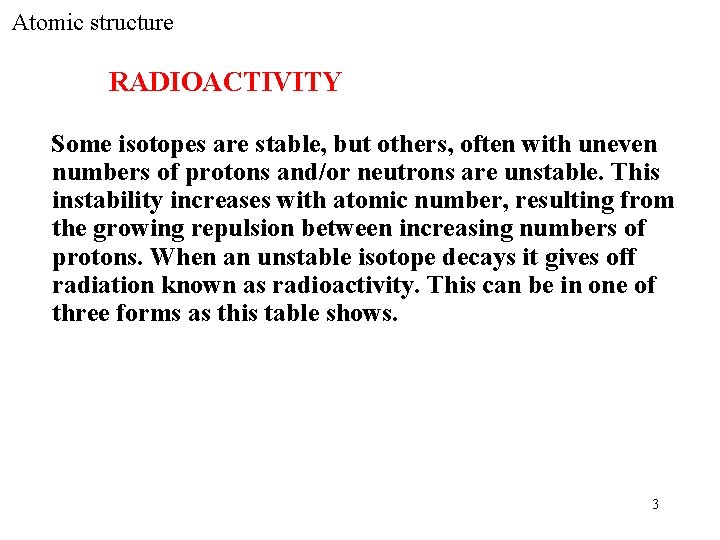
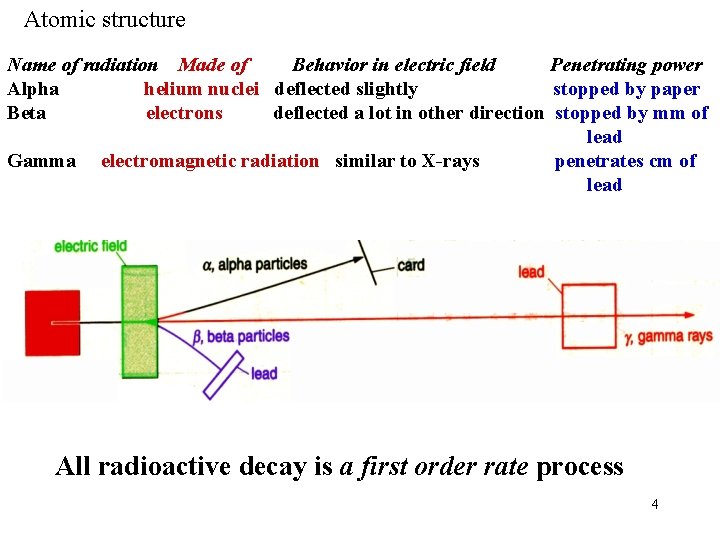
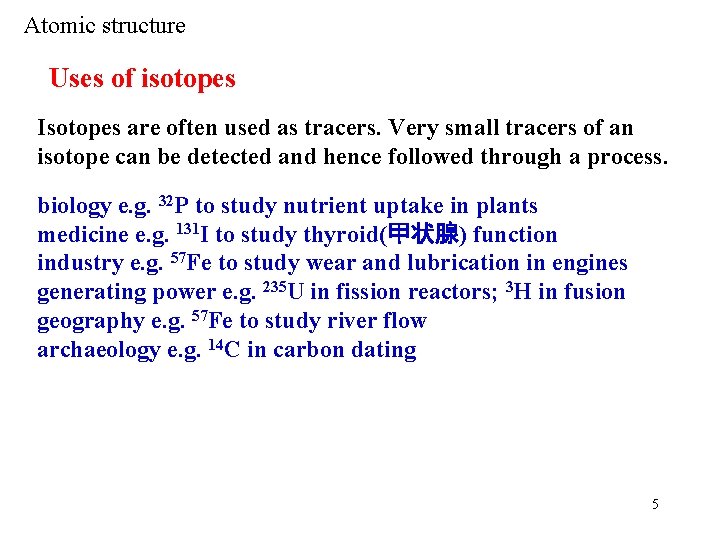
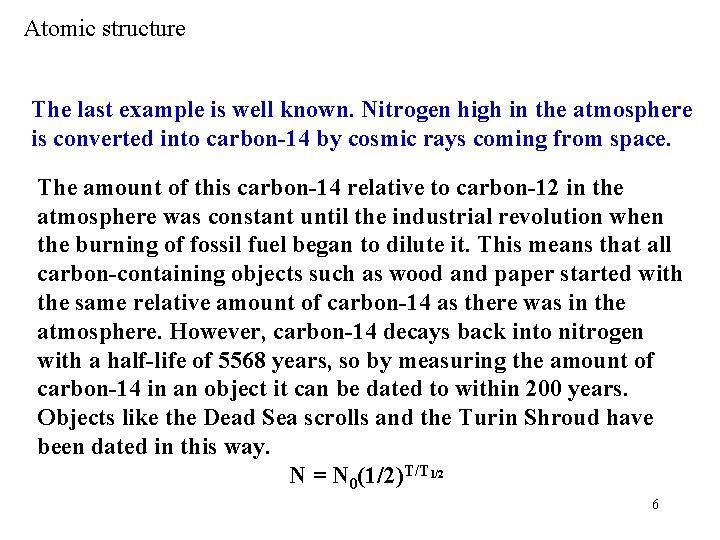
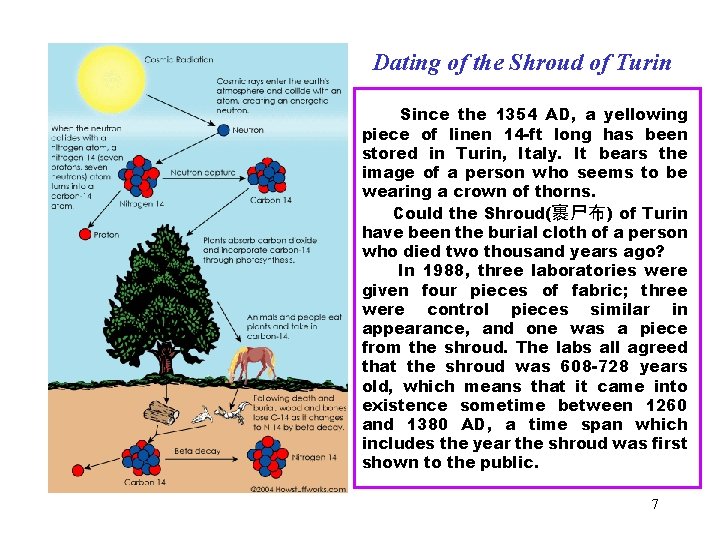
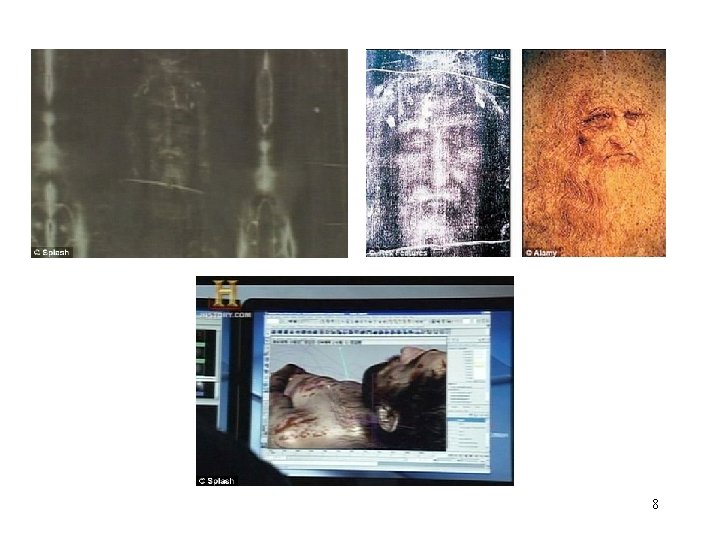
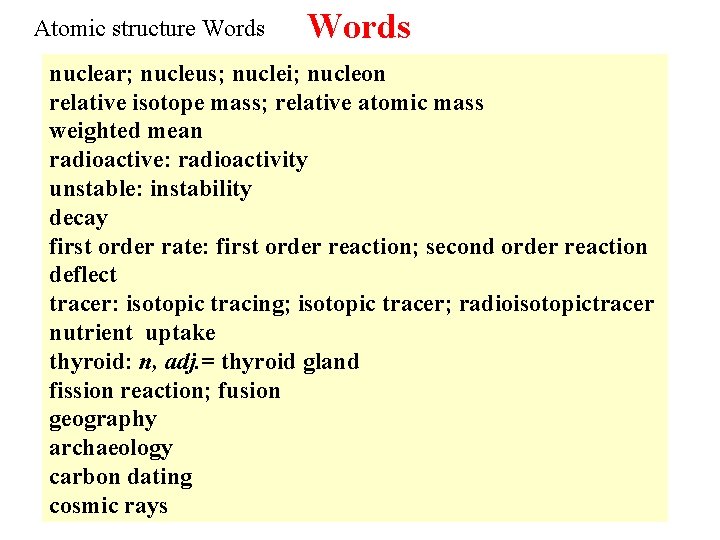
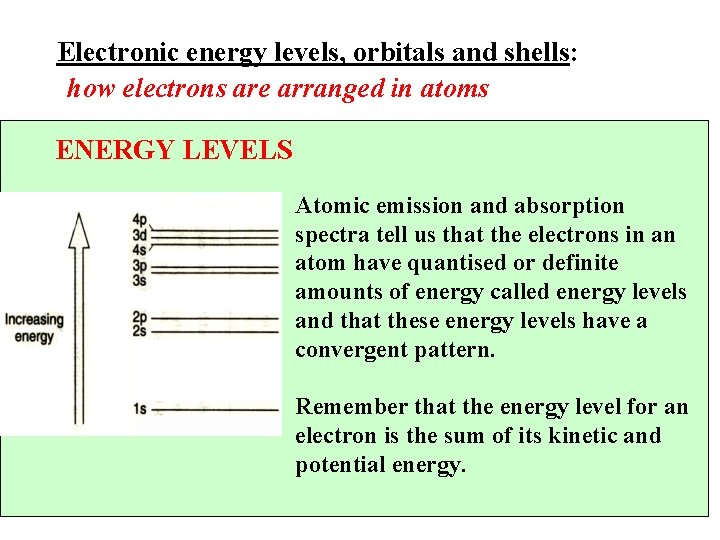
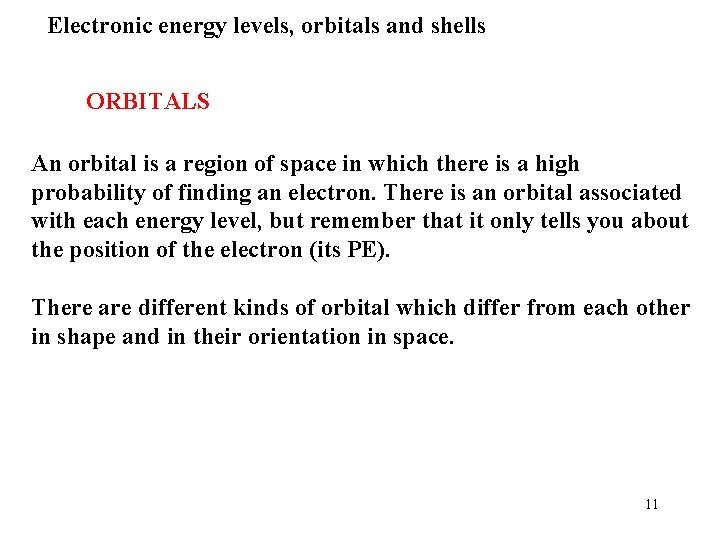
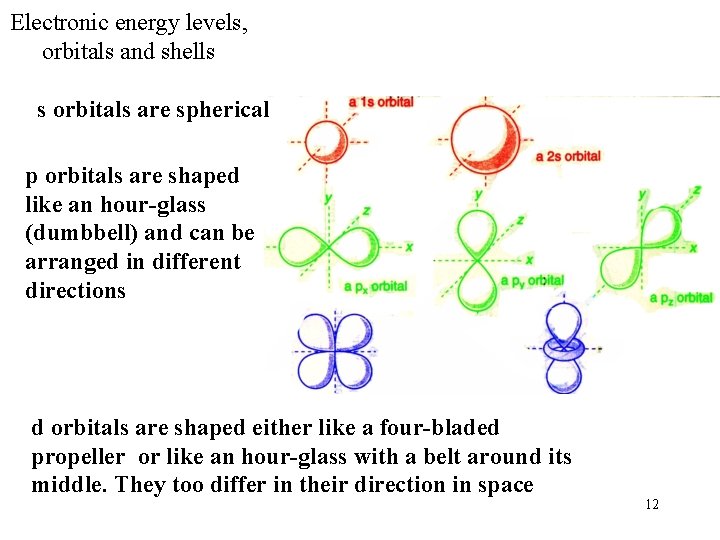
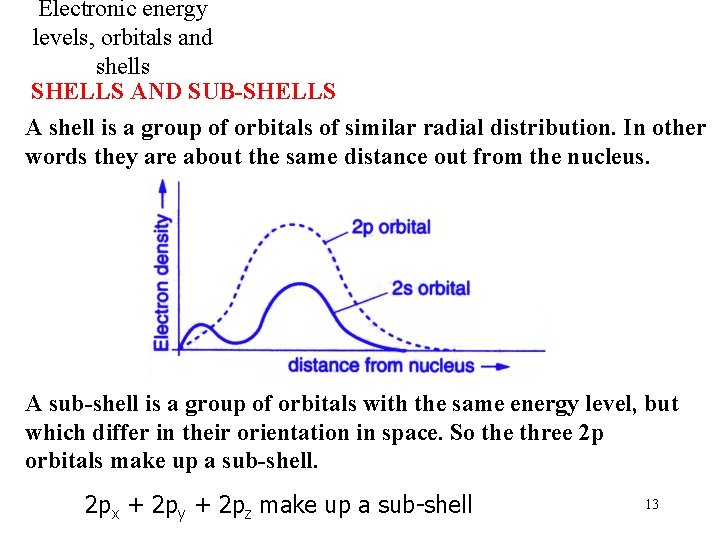
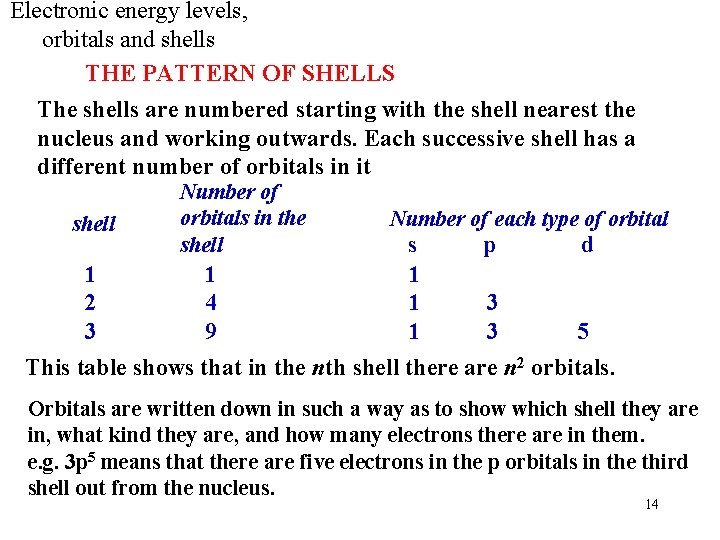
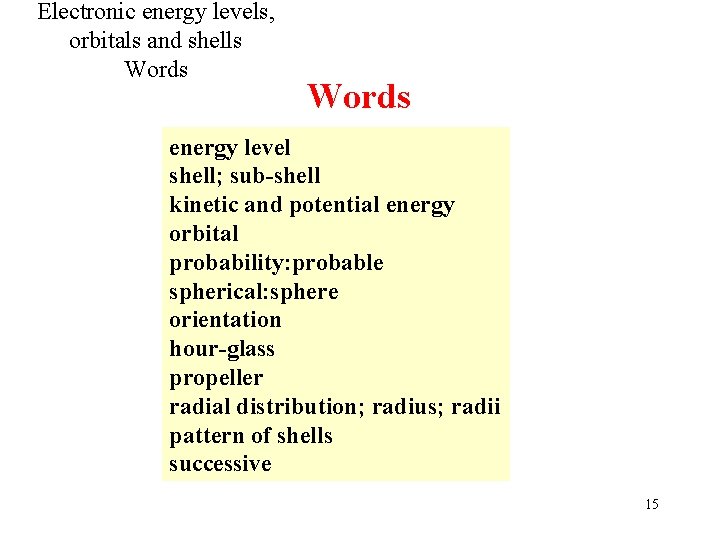
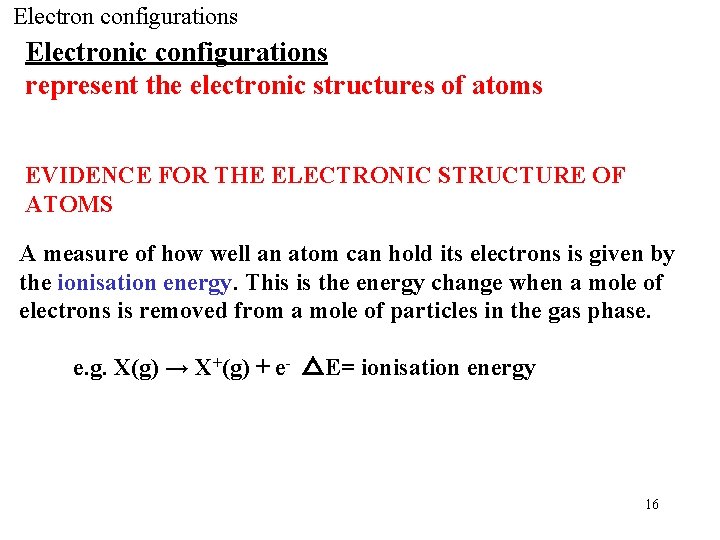
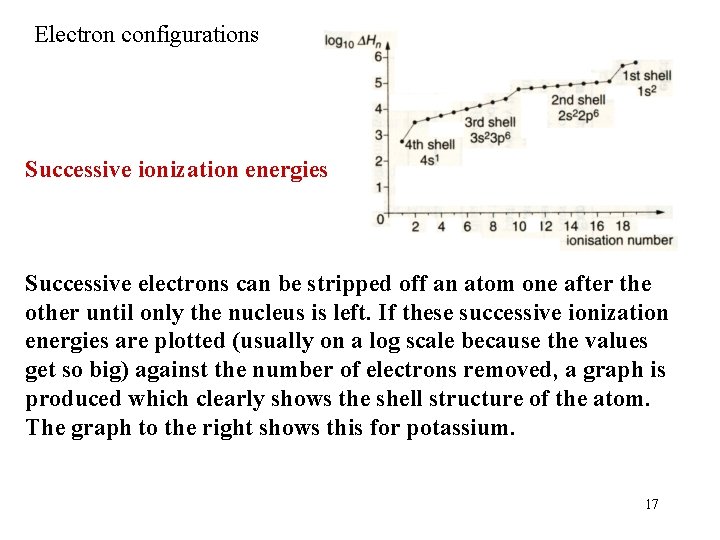
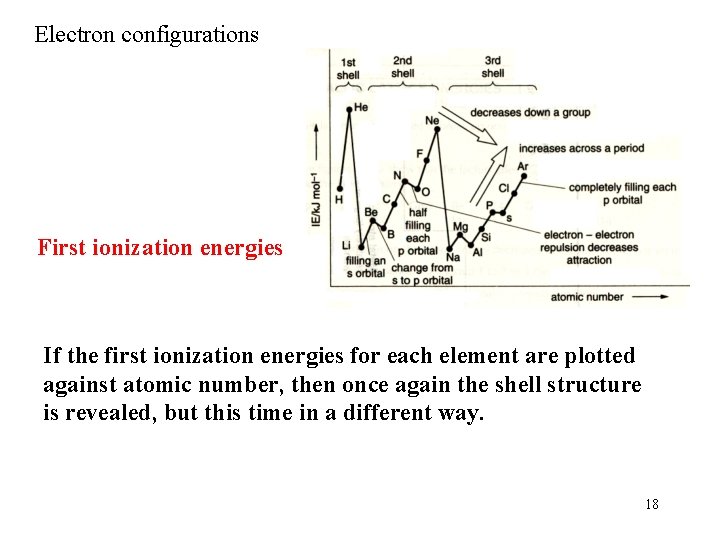
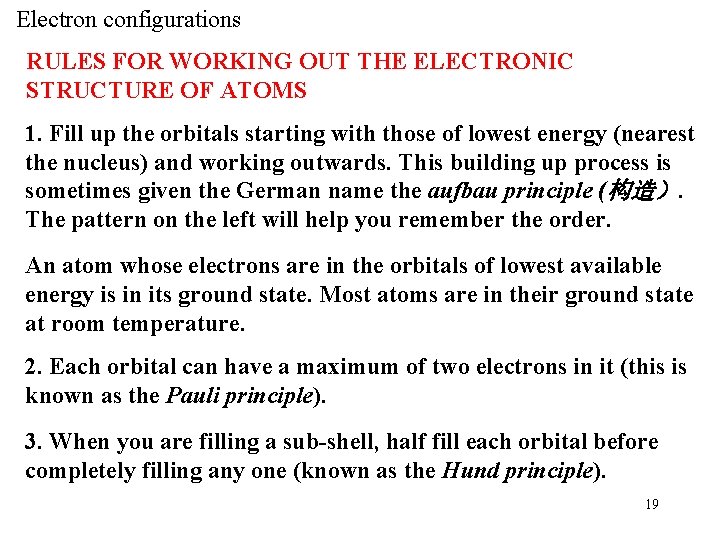
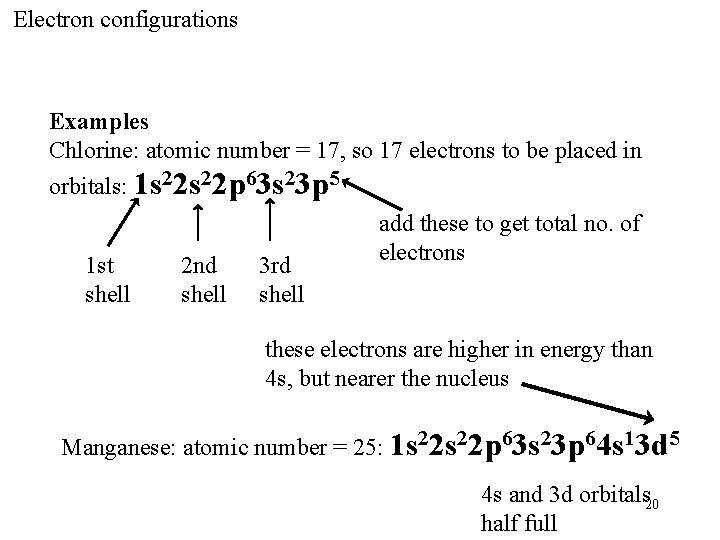
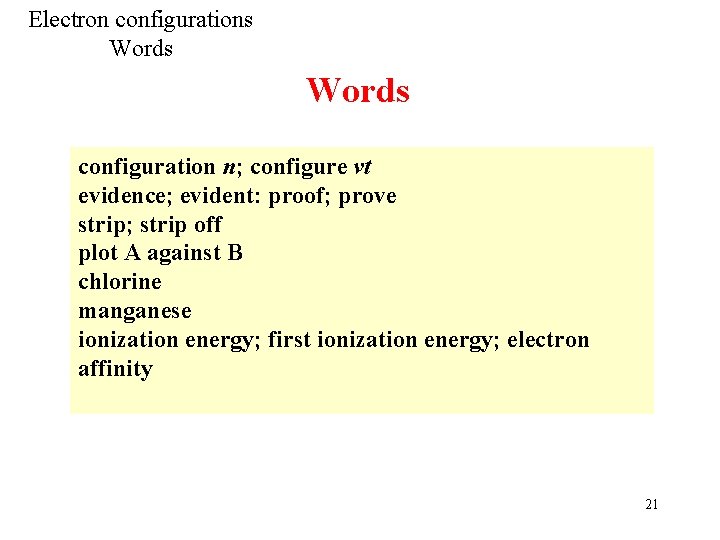
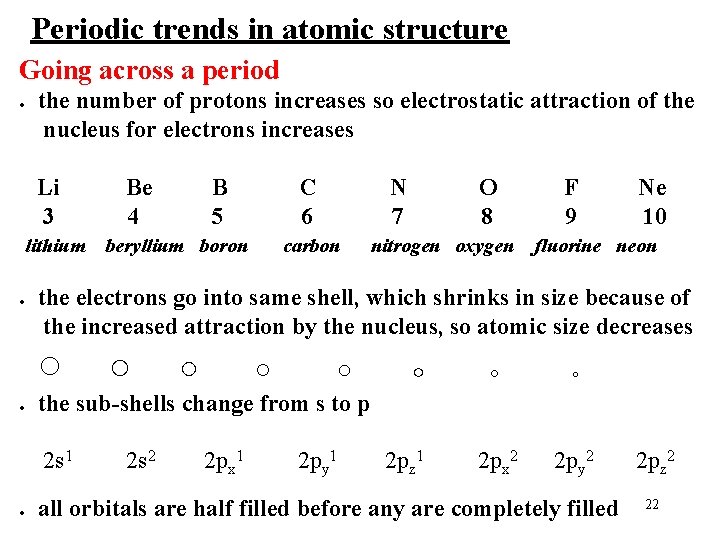
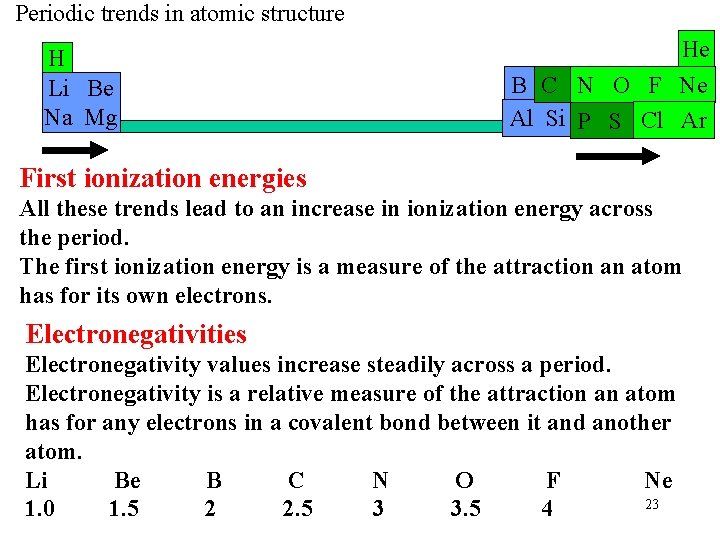
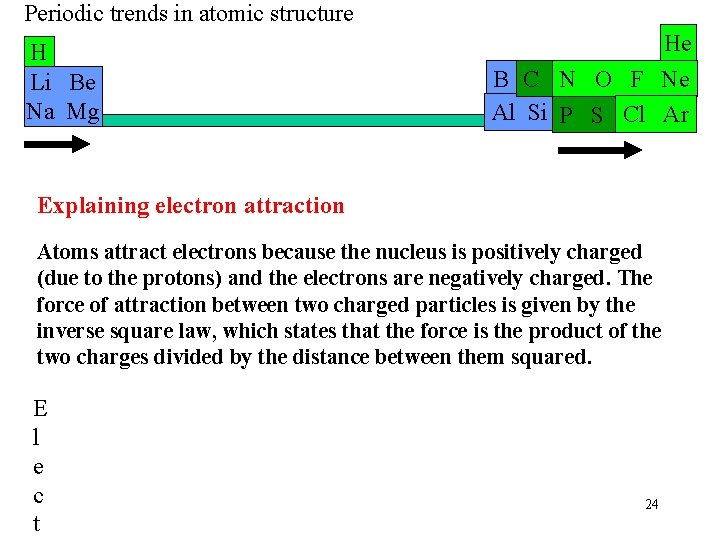
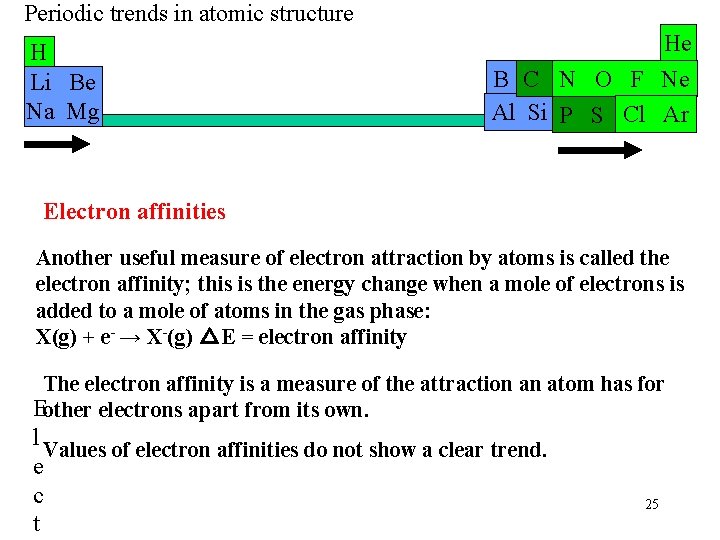
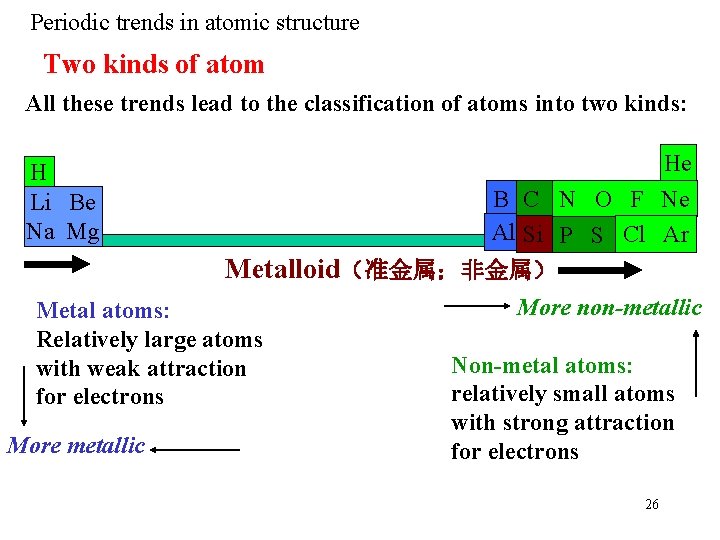
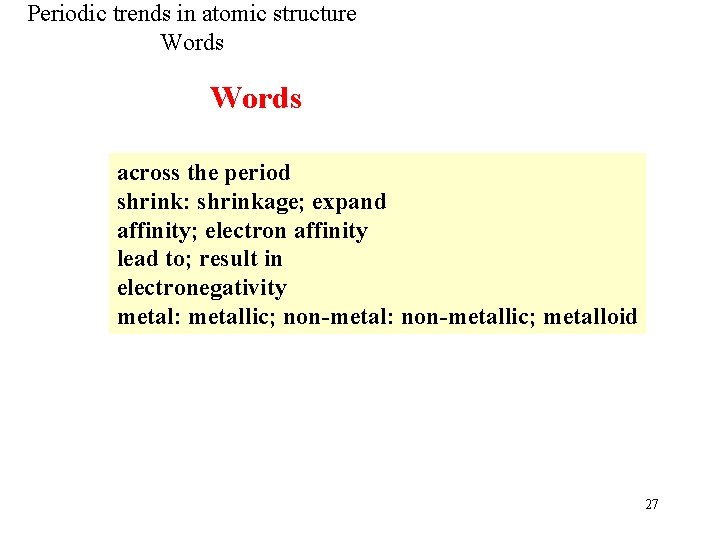
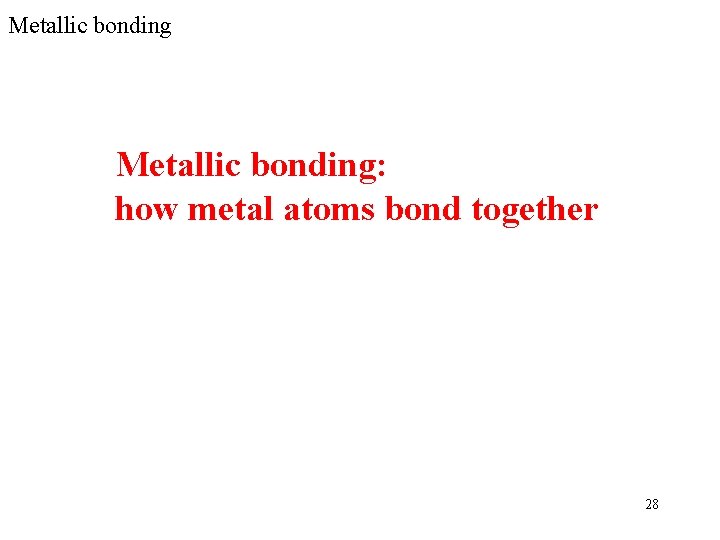
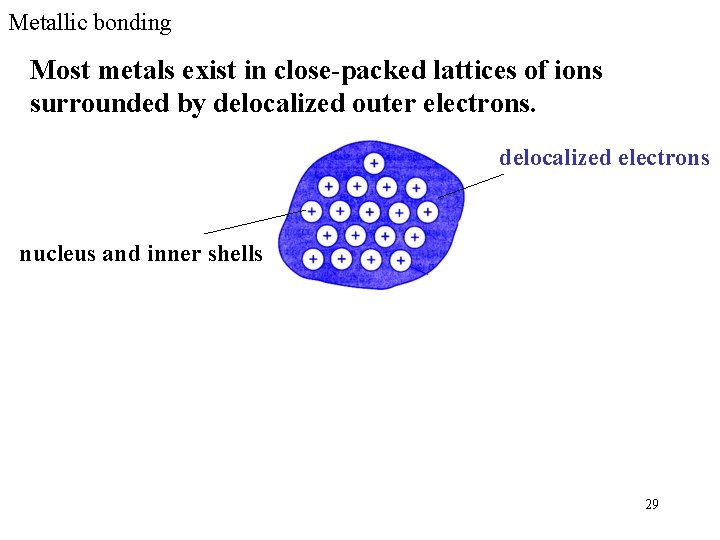
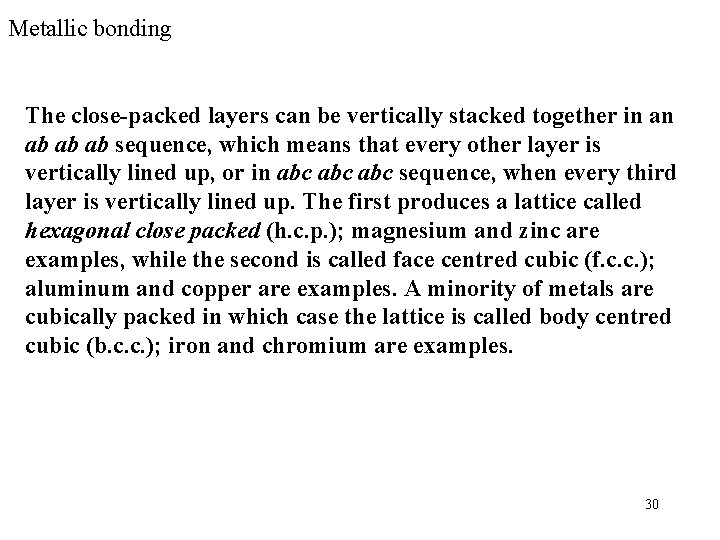
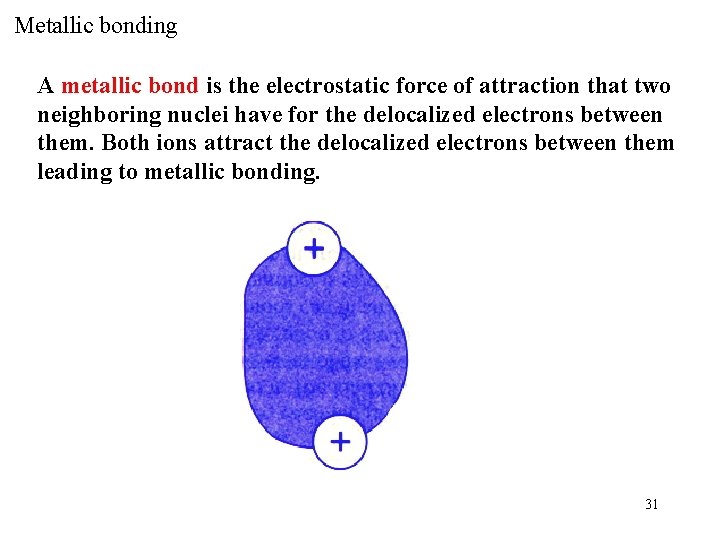
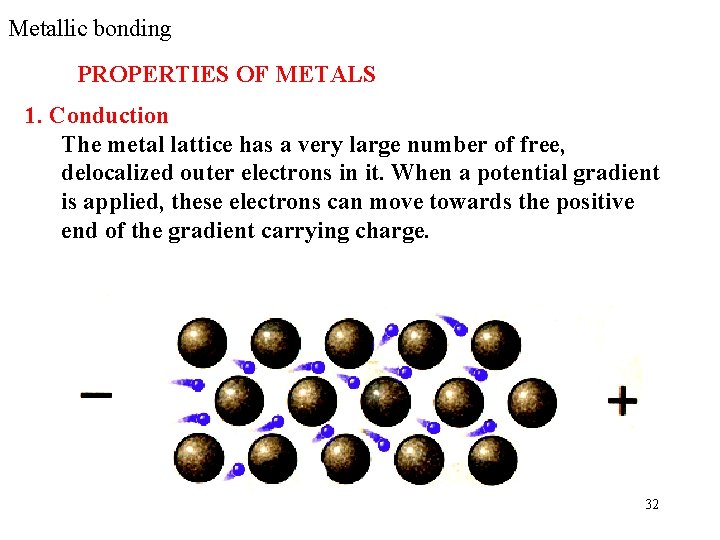
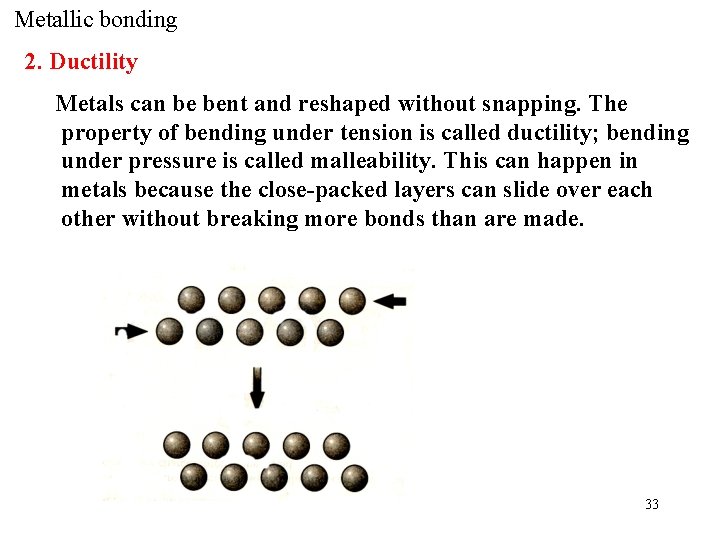
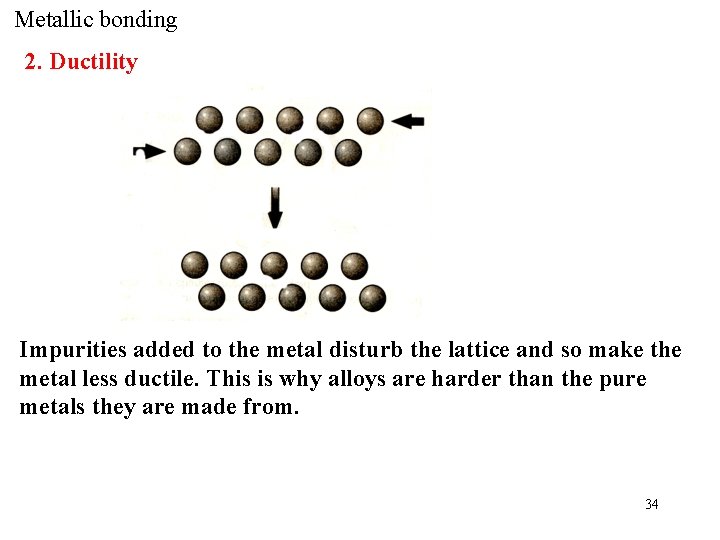
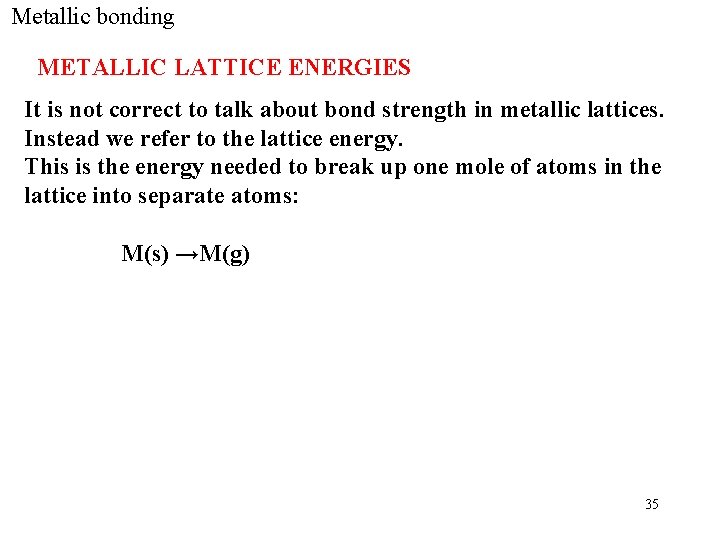
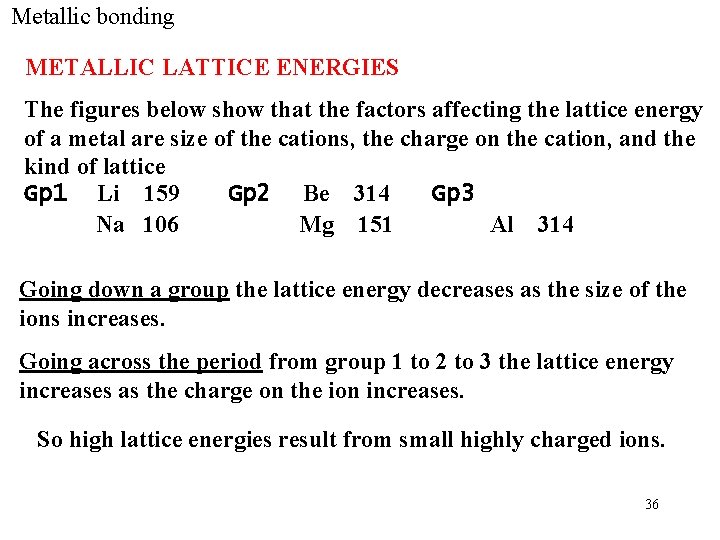
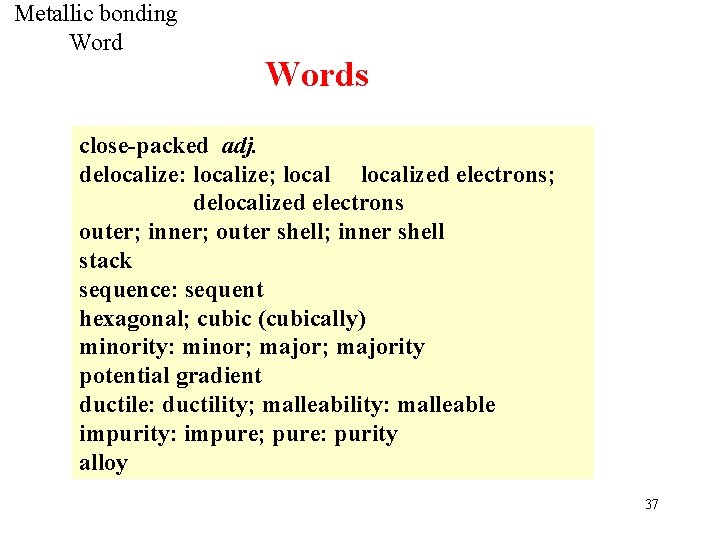
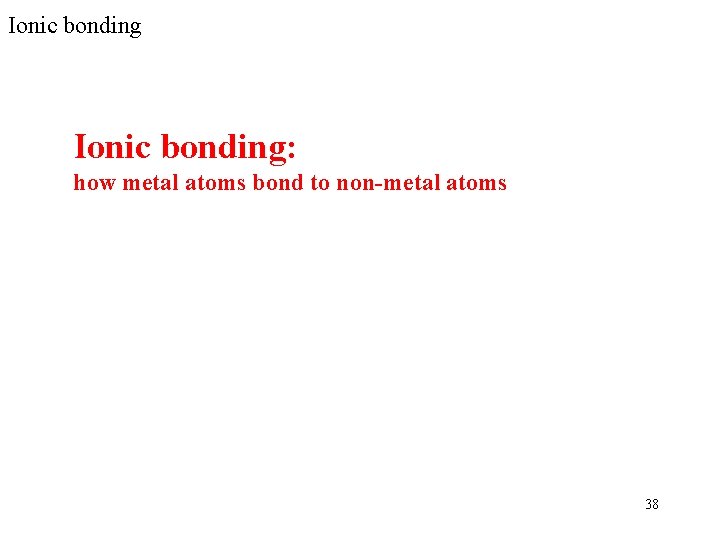
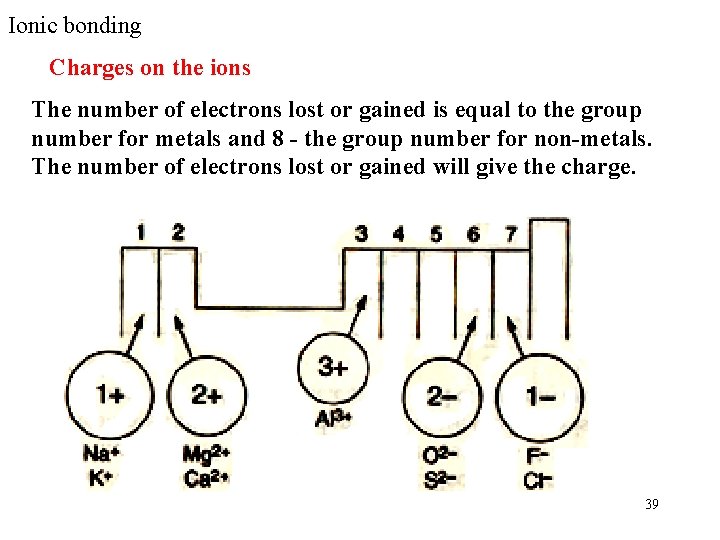
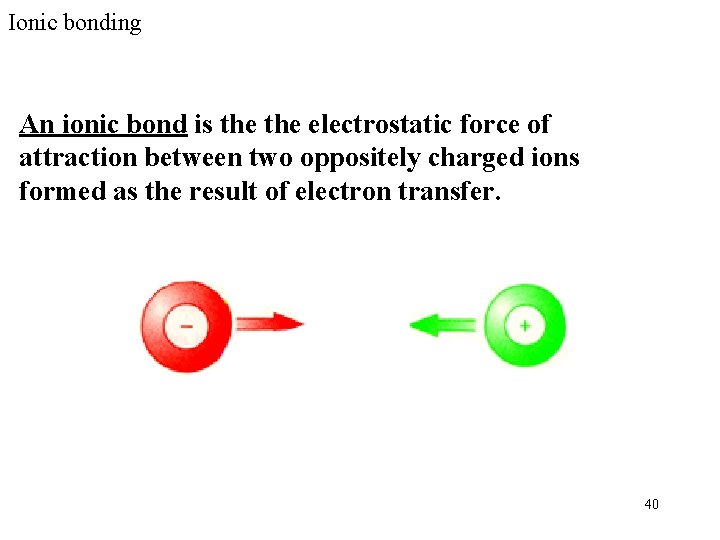
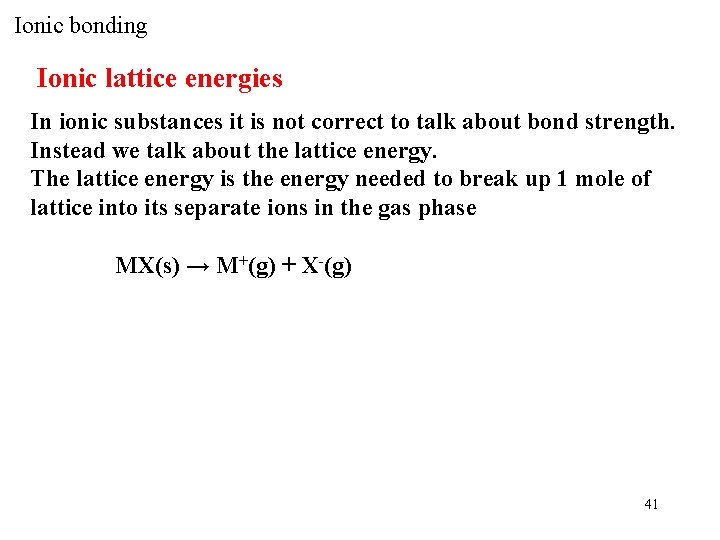
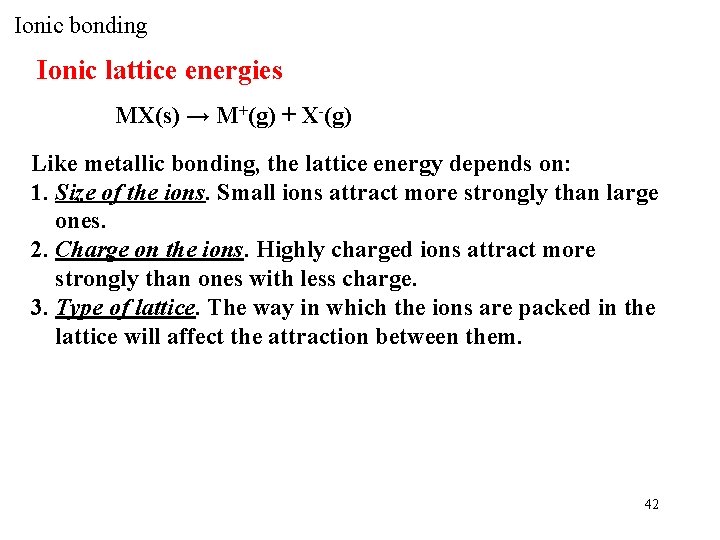
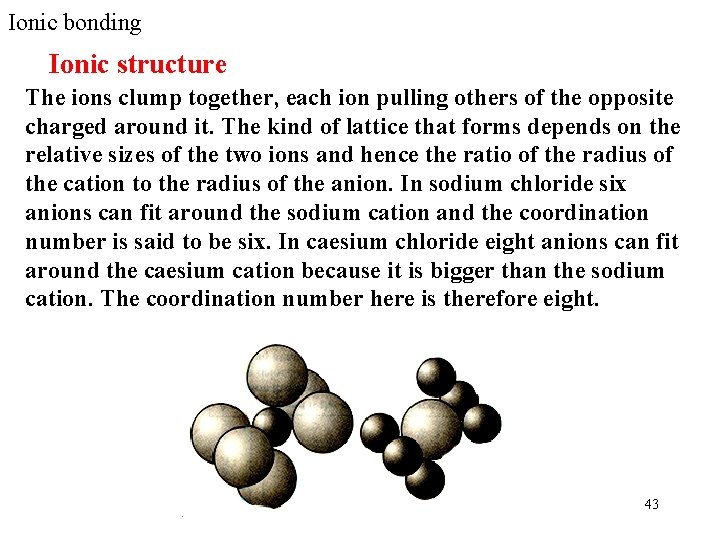
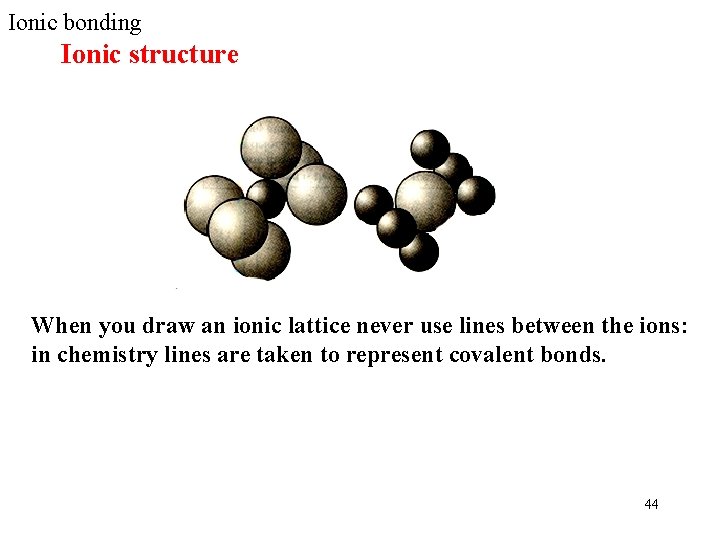
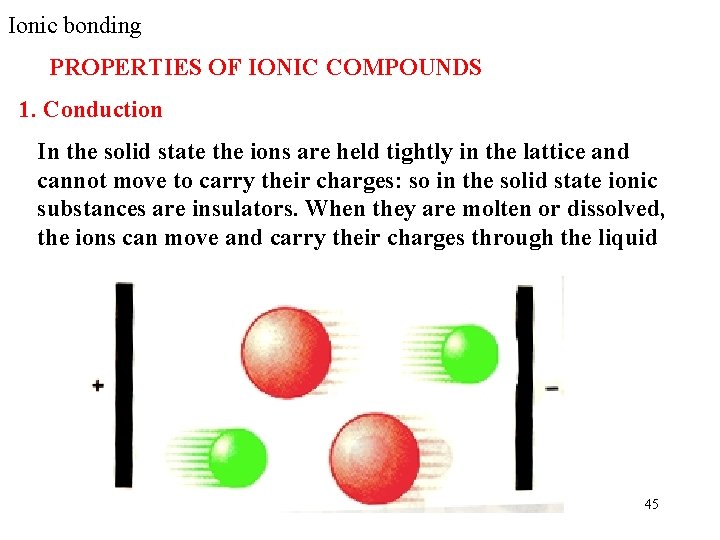
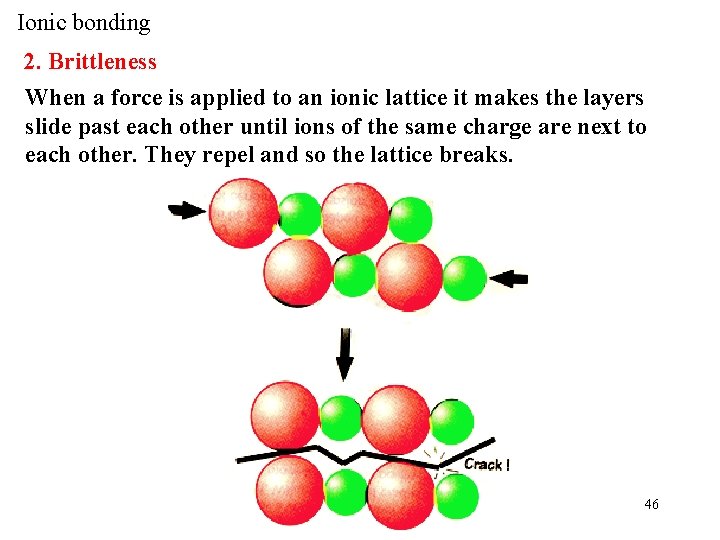
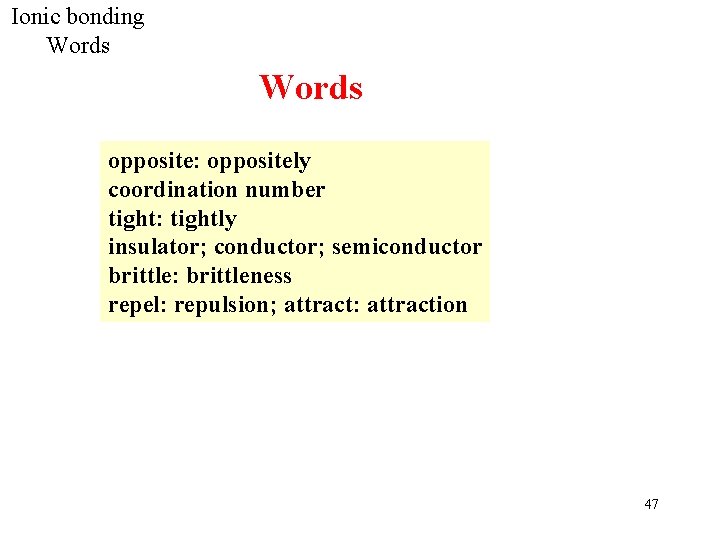
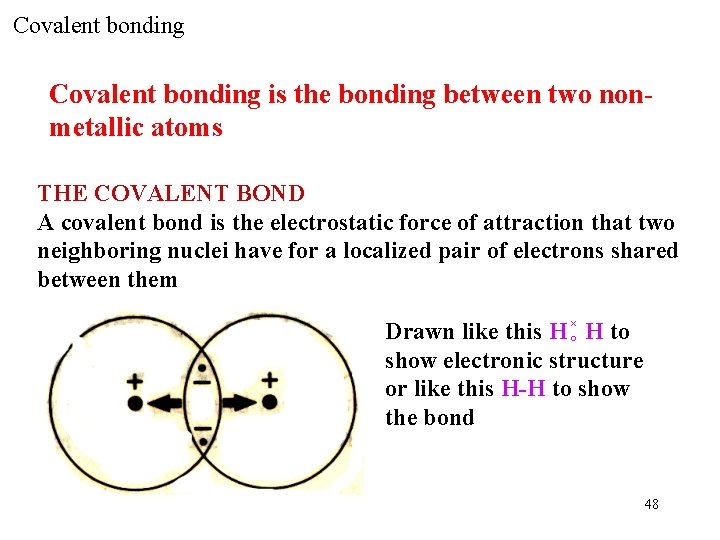
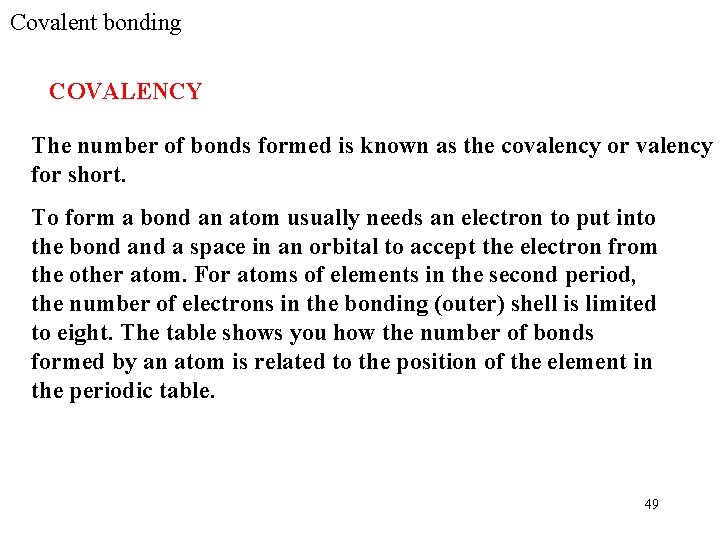
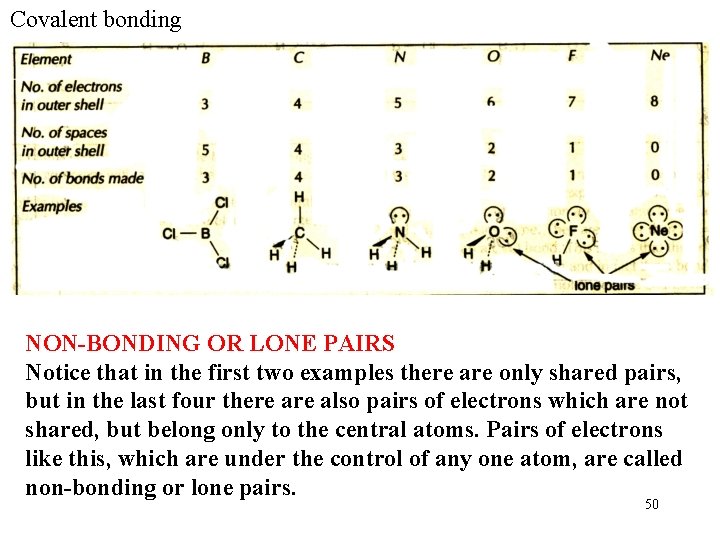
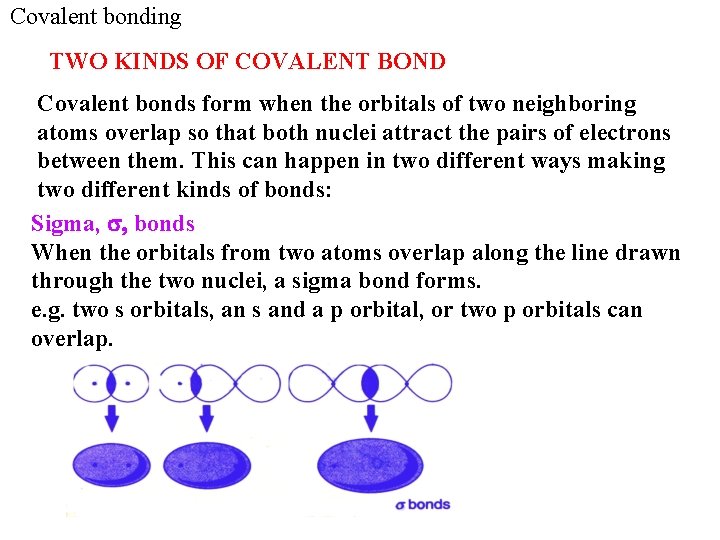
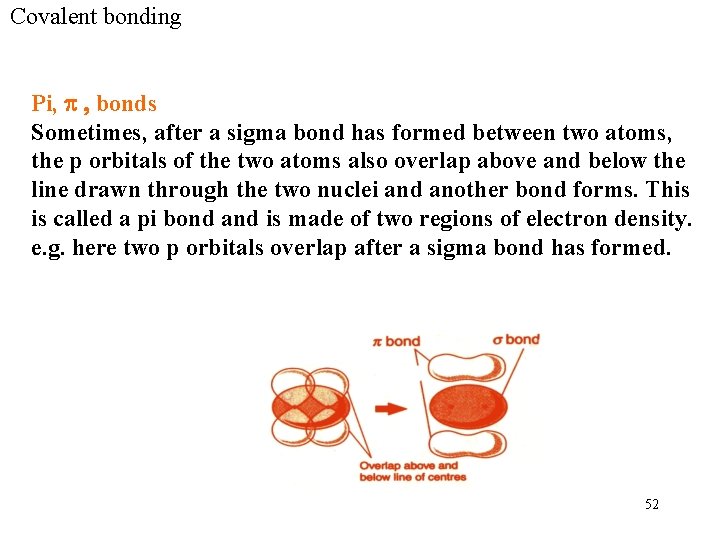
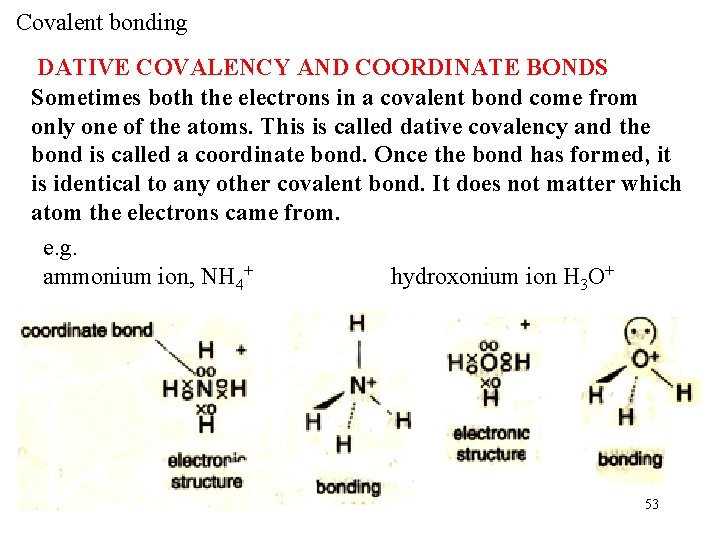
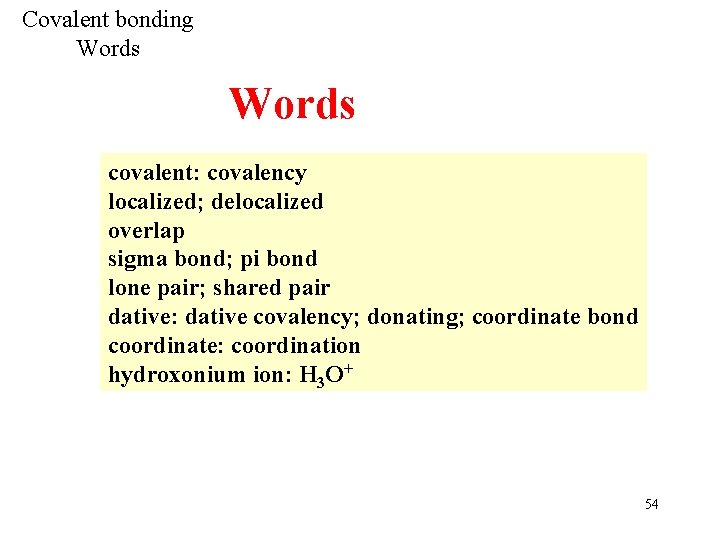
![Covalent bonding Quadruple bond K 2[Re 2 Cl 8]·H 2 O * * * Covalent bonding Quadruple bond K 2[Re 2 Cl 8]·H 2 O * * *](https://slidetodoc.com/presentation_image_h2/02c9dfa52b012cef7f984c73b1b96738/image-55.jpg)
- Slides: 55
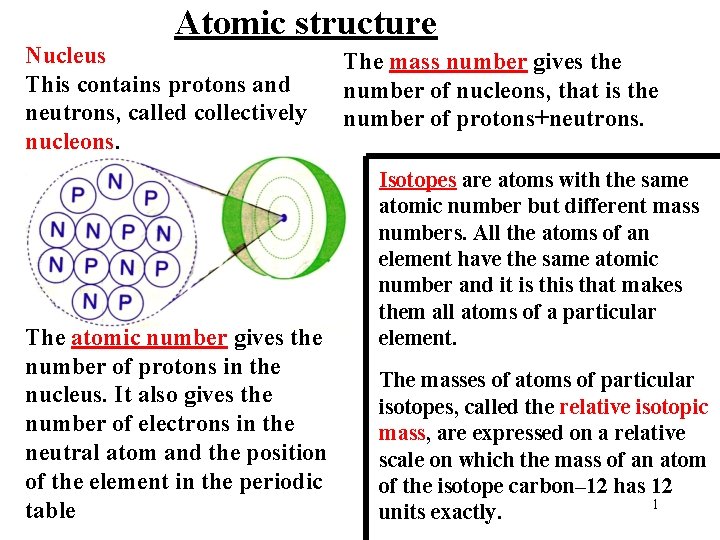
Atomic structure Nucleus This contains protons and neutrons, called collectively nucleons. The atomic number gives the number of protons in the nucleus. It also gives the number of electrons in the neutral atom and the position of the element in the periodic table The mass number gives the number of nucleons, that is the number of protons+neutrons. Isotopes are atoms with the same atomic number but different mass numbers. All the atoms of an element have the same atomic number and it is that makes them all atoms of a particular element. The masses of atoms of particular isotopes, called the relative isotopic mass, are expressed on a relative scale on which the mass of an atom of the isotope carbon– 12 has 12 1 units exactly.
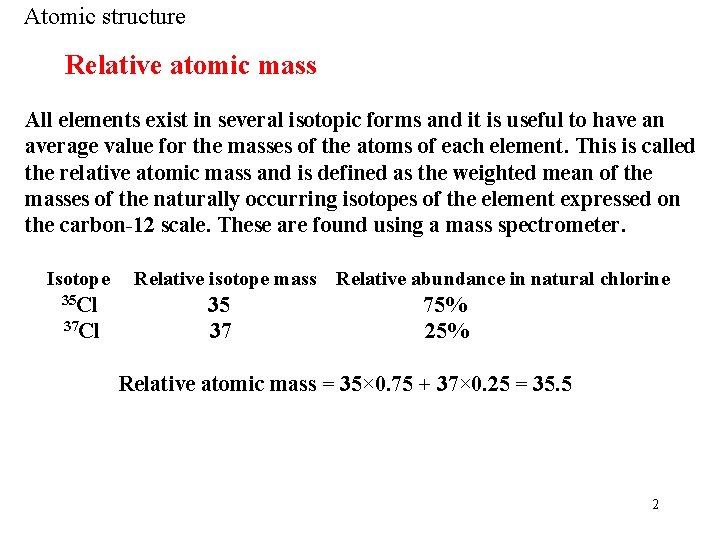
Atomic structure Relative atomic mass All elements exist in several isotopic forms and it is useful to have an average value for the masses of the atoms of each element. This is called the relative atomic mass and is defined as the weighted mean of the masses of the naturally occurring isotopes of the element expressed on the carbon-12 scale. These are found using a mass spectrometer. Isotope 35 Cl 37 Cl Relative isotope mass 35 37 Relative abundance in natural chlorine 75% 25% Relative atomic mass = 35× 0. 75 + 37× 0. 25 = 35. 5 2
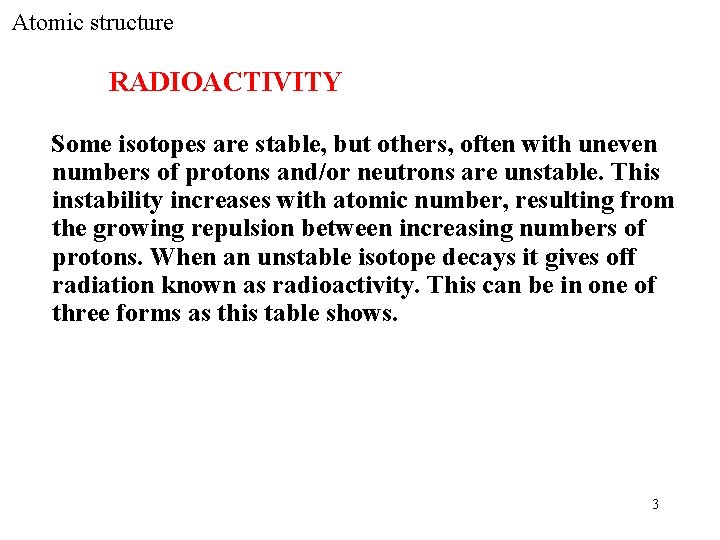
Atomic structure RADIOACTIVITY Some isotopes are stable, but others, often with uneven numbers of protons and/or neutrons are unstable. This instability increases with atomic number, resulting from the growing repulsion between increasing numbers of protons. When an unstable isotope decays it gives off radiation known as radioactivity. This can be in one of three forms as this table shows. 3
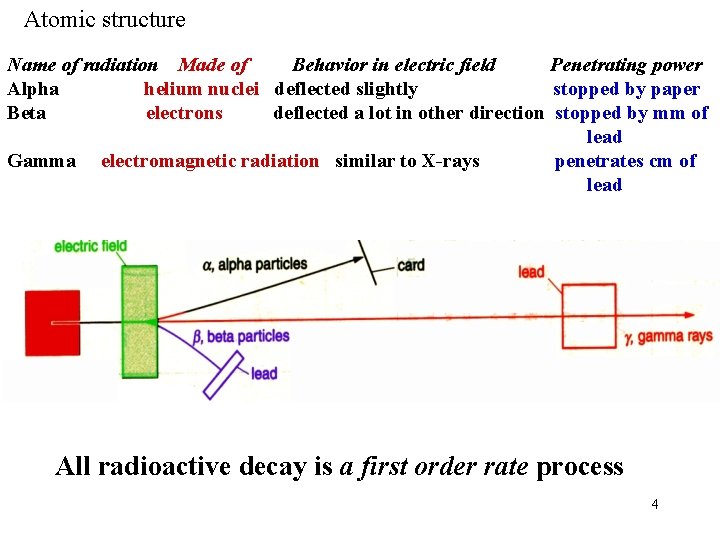
Atomic structure Name of radiation Made of Behavior in electric field Penetrating power Alpha helium nuclei deflected slightly stopped by paper Beta electrons deflected a lot in other direction stopped by mm of lead Gamma electromagnetic radiation similar to X-rays penetrates cm of lead All radioactive decay is a first order rate process 4
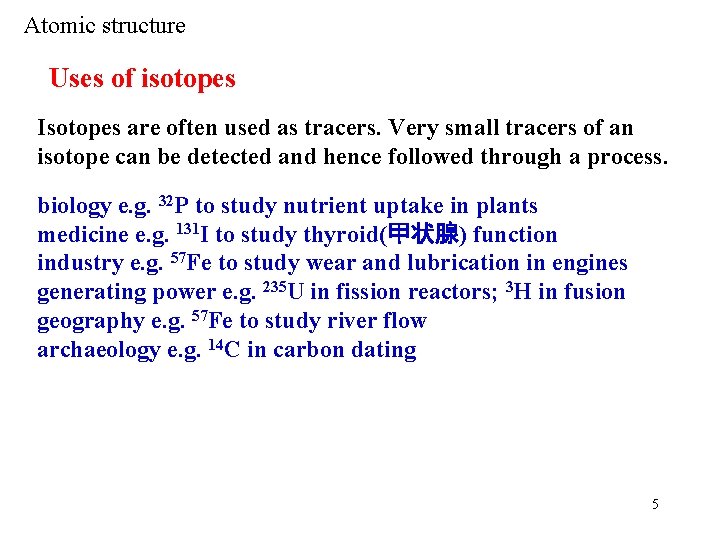
Atomic structure Uses of isotopes Isotopes are often used as tracers. Very small tracers of an isotope can be detected and hence followed through a process. biology e. g. 32 P to study nutrient uptake in plants medicine e. g. 131 I to study thyroid(甲状腺) function industry e. g. 57 Fe to study wear and lubrication in engines generating power e. g. 235 U in fission reactors; 3 H in fusion geography e. g. 57 Fe to study river flow archaeology e. g. 14 C in carbon dating 5
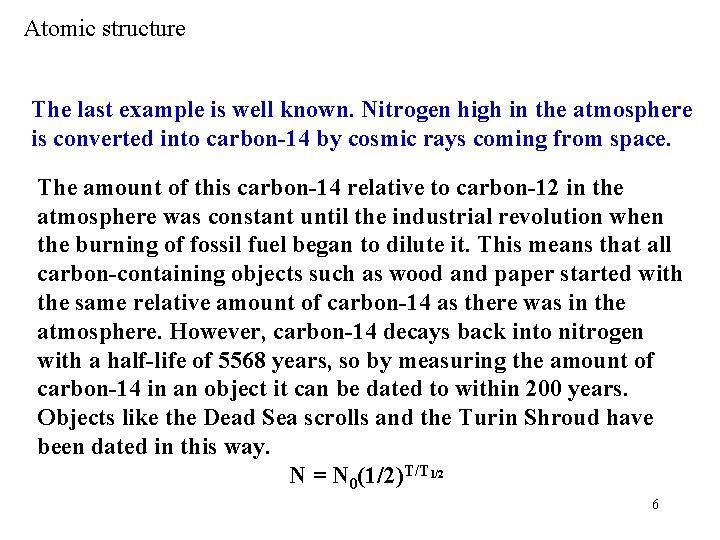
Atomic structure The last example is well known. Nitrogen high in the atmosphere is converted into carbon-14 by cosmic rays coming from space. The amount of this carbon-14 relative to carbon-12 in the atmosphere was constant until the industrial revolution when the burning of fossil fuel began to dilute it. This means that all carbon-containing objects such as wood and paper started with the same relative amount of carbon-14 as there was in the atmosphere. However, carbon-14 decays back into nitrogen with a half-life of 5568 years, so by measuring the amount of carbon-14 in an object it can be dated to within 200 years. Objects like the Dead Sea scrolls and the Turin Shroud have been dated in this way. N = N 0(1/2)T/T 1/2 6
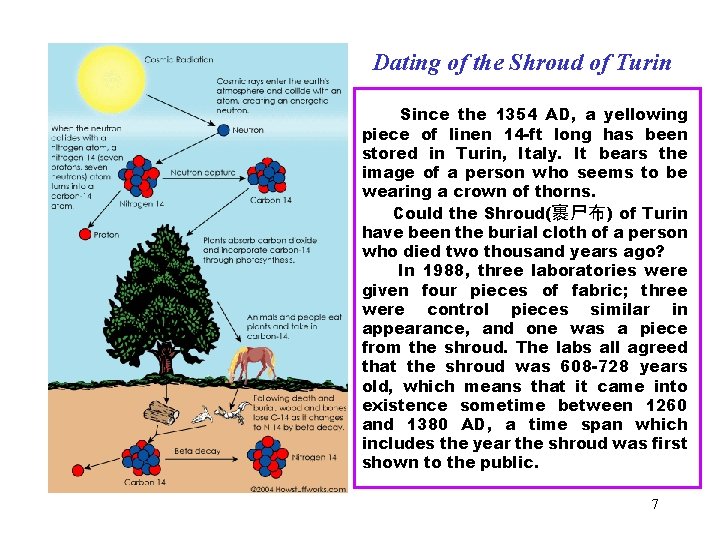
Dating of the Shroud of Turin Since the 1354 AD, a yellowing piece of linen 14 -ft long has been stored in Turin, Italy. It bears the image of a person who seems to be wearing a crown of thorns. Could the Shroud(裹尸布) of Turin have been the burial cloth of a person who died two thousand years ago? In 1988, three laboratories were given four pieces of fabric; three were control pieces similar in appearance, and one was a piece from the shroud. The labs all agreed that the shroud was 608 -728 years old, which means that it came into existence sometime between 1260 and 1380 AD, a time span which includes the year the shroud was first shown to the public. 7
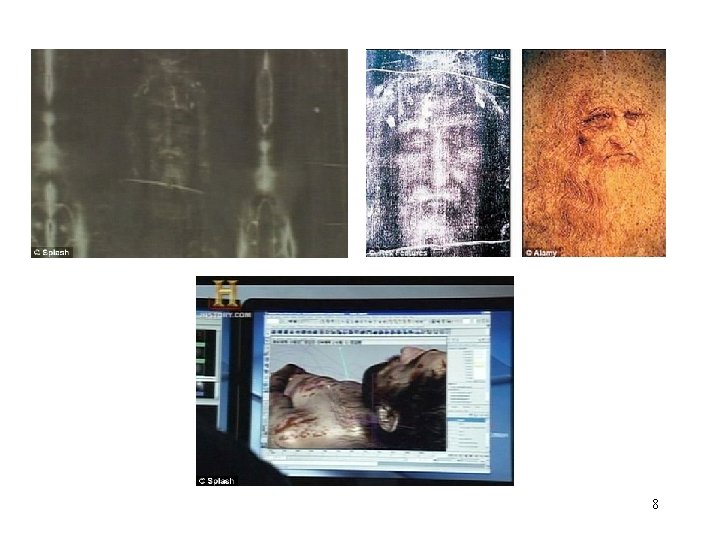
8
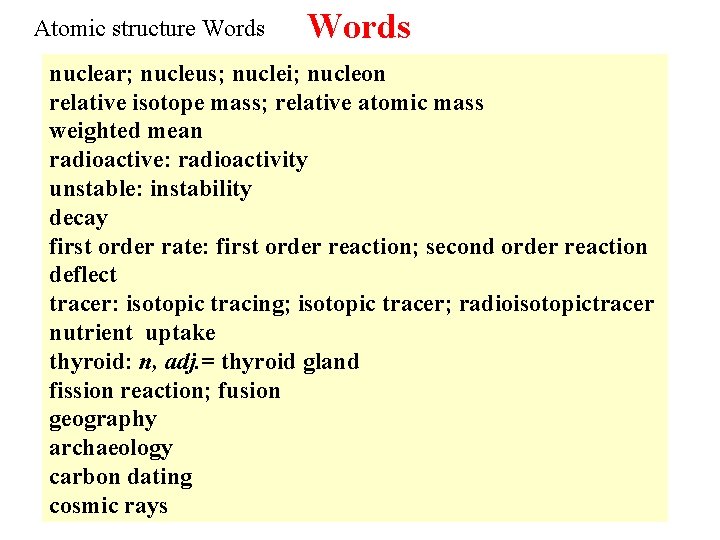
Atomic structure Words nuclear; nucleus; nuclei; nucleon relative isotope mass; relative atomic mass weighted mean radioactive: radioactivity unstable: instability decay first order rate: first order reaction; second order reaction deflect tracer: isotopic tracing; isotopic tracer; radioisotopictracer nutrient uptake thyroid: n, adj. = thyroid gland fission reaction; fusion geography archaeology carbon dating 9 cosmic rays
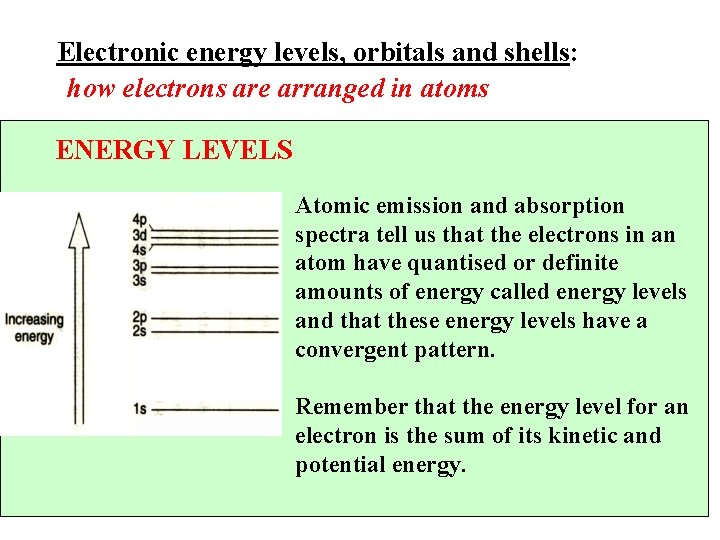
Electronic energy levels, orbitals and shells: how electrons are arranged in atoms ENERGY LEVELS Atomic emission and absorption spectra tell us that the electrons in an atom have quantised or definite amounts of energy called energy levels and that these energy levels have a convergent pattern. Remember that the energy level for an electron is the sum of its kinetic and potential energy. 10
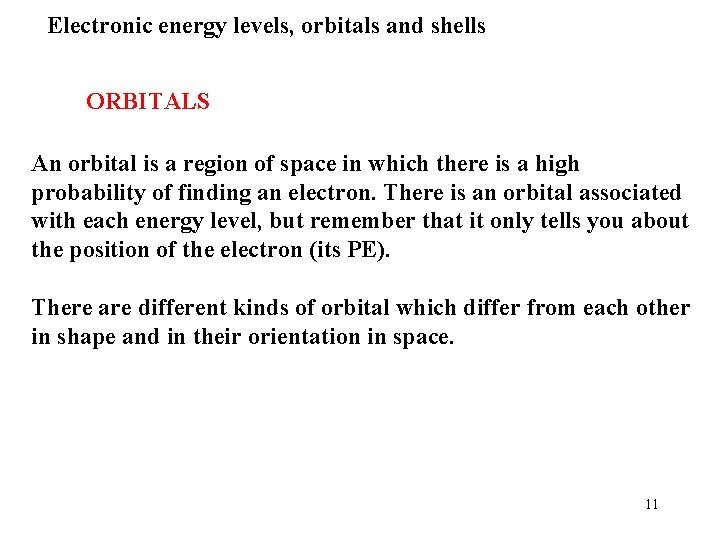
Electronic energy levels, orbitals and shells ORBITALS An orbital is a region of space in which there is a high probability of finding an electron. There is an orbital associated with each energy level, but remember that it only tells you about the position of the electron (its PE). There are different kinds of orbital which differ from each other in shape and in their orientation in space. 11
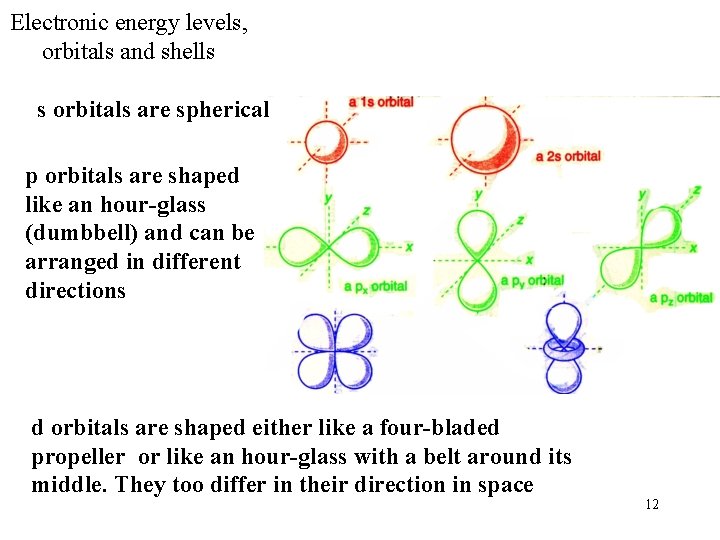
Electronic energy levels, orbitals and shells s orbitals are spherical p orbitals are shaped like an hour-glass (dumbbell) and can be arranged in different directions d orbitals are shaped either like a four-bladed propeller or like an hour-glass with a belt around its middle. They too differ in their direction in space 12
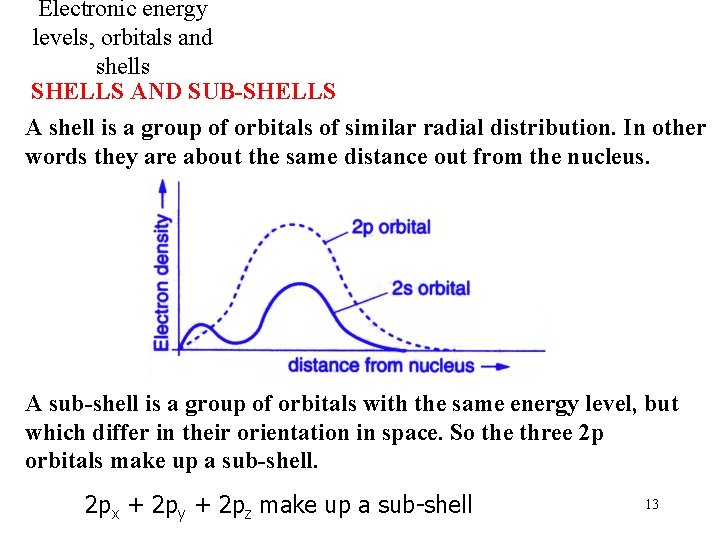
Electronic energy levels, orbitals and shells SHELLS AND SUB-SHELLS A shell is a group of orbitals of similar radial distribution. In other words they are about the same distance out from the nucleus. A sub-shell is a group of orbitals with the same energy level, but which differ in their orientation in space. So the three 2 p orbitals make up a sub-shell. 2 px + 2 py + 2 pz make up a sub-shell 13
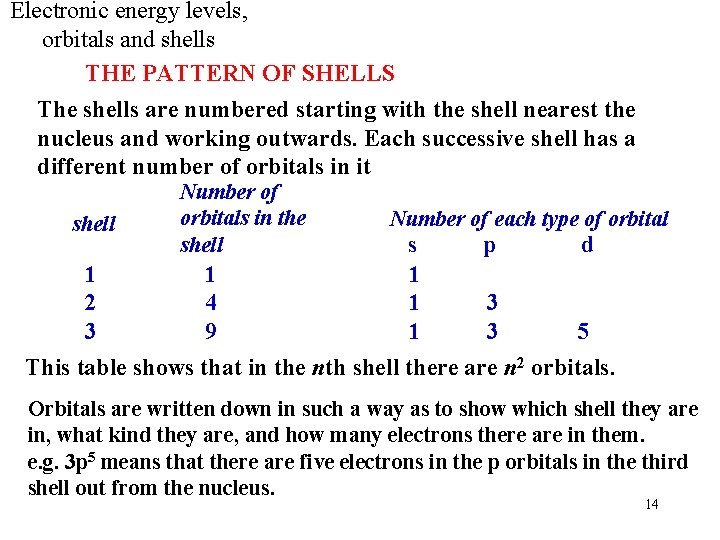
Electronic energy levels, orbitals and shells THE PATTERN OF SHELLS The shells are numbered starting with the shell nearest the nucleus and working outwards. Each successive shell has a different number of orbitals in it shell Number of orbitals in the shell Number of each type of orbital s p d 1 1 1 2 4 1 3 3 9 1 3 5 This table shows that in the nth shell there are n 2 orbitals. Orbitals are written down in such a way as to show which shell they are in, what kind they are, and how many electrons there are in them. e. g. 3 p 5 means that there are five electrons in the p orbitals in the third shell out from the nucleus. 14
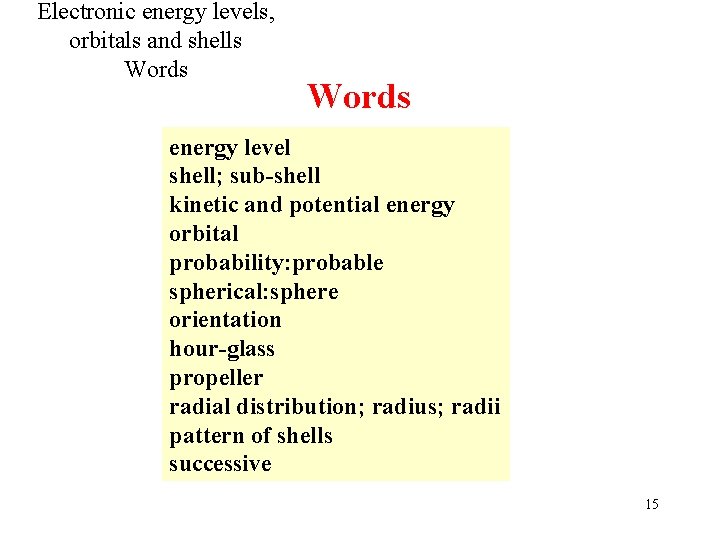
Electronic energy levels, orbitals and shells Words energy level shell; sub-shell kinetic and potential energy orbital probability: probable spherical: sphere orientation hour-glass propeller radial distribution; radius; radii pattern of shells successive 15
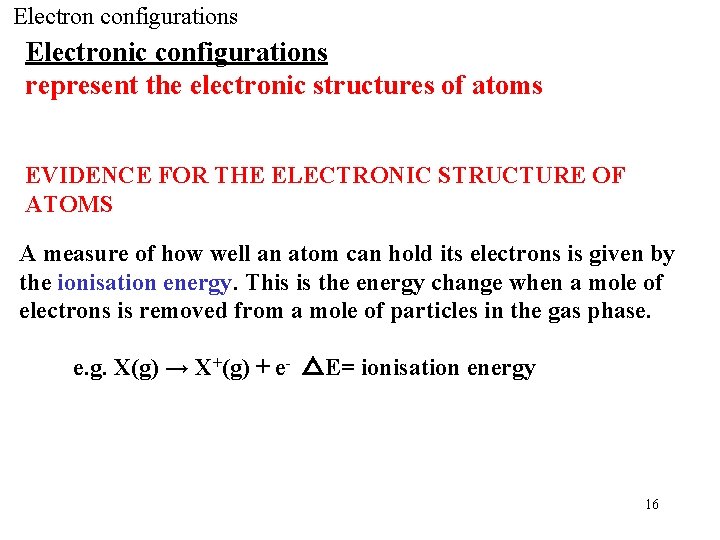
Electron configurations Electronic configurations represent the electronic structures of atoms EVIDENCE FOR THE ELECTRONIC STRUCTURE OF ATOMS A measure of how well an atom can hold its electrons is given by the ionisation energy. This is the energy change when a mole of electrons is removed from a mole of particles in the gas phase. e. g. X(g) → X+(g) + e- △E= ionisation energy 16
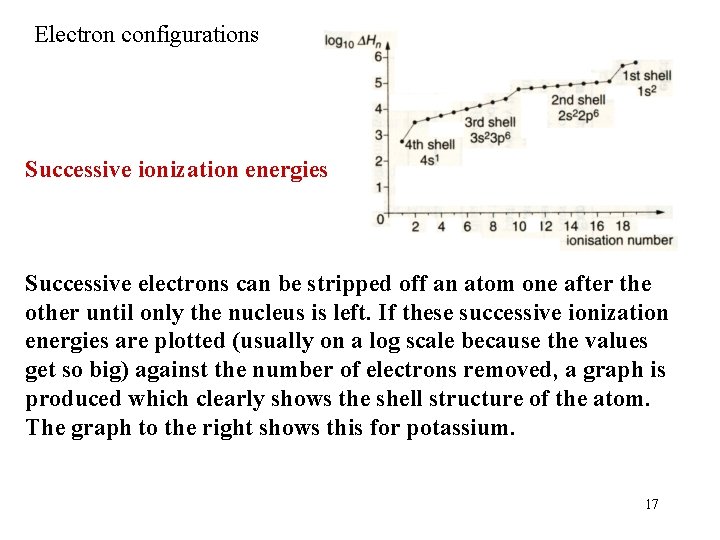
Electron configurations Successive ionization energies Successive electrons can be stripped off an atom one after the other until only the nucleus is left. If these successive ionization energies are plotted (usually on a log scale because the values get so big) against the number of electrons removed, a graph is produced which clearly shows the shell structure of the atom. The graph to the right shows this for potassium. 17
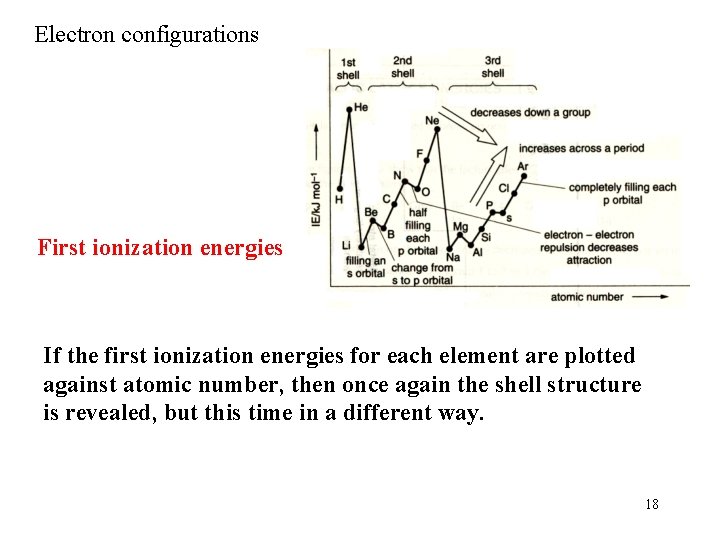
Electron configurations First ionization energies If the first ionization energies for each element are plotted against atomic number, then once again the shell structure is revealed, but this time in a different way. 18
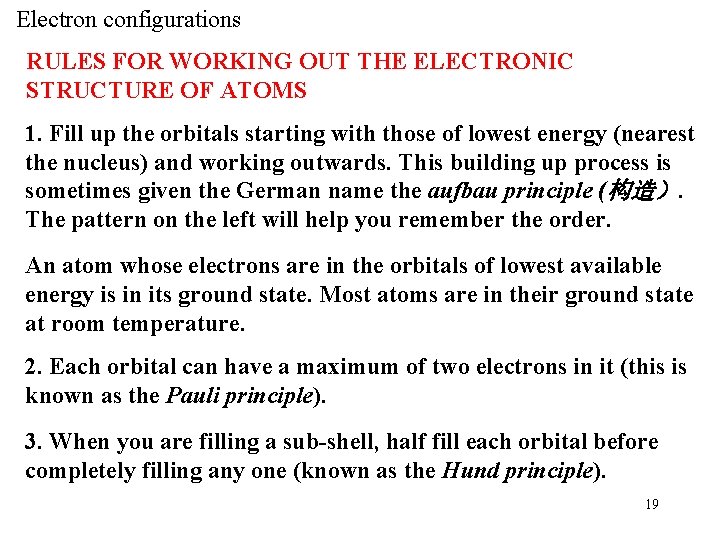
Electron configurations RULES FOR WORKING OUT THE ELECTRONIC STRUCTURE OF ATOMS 1. Fill up the orbitals starting with those of lowest energy (nearest the nucleus) and working outwards. This building up process is sometimes given the German name the aufbau principle (构造). The pattern on the left will help you remember the order. An atom whose electrons are in the orbitals of lowest available energy is in its ground state. Most atoms are in their ground state at room temperature. 2. Each orbital can have a maximum of two electrons in it (this is known as the Pauli principle). 3. When you are filling a sub-shell, half fill each orbital before completely filling any one (known as the Hund principle). 19
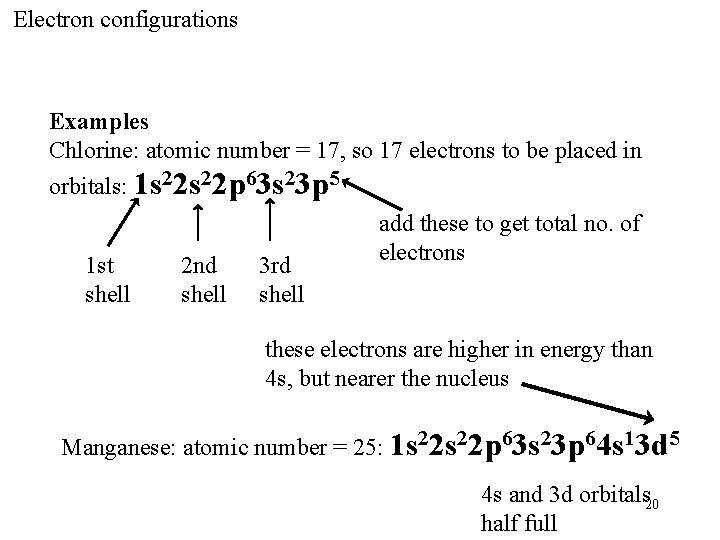
Electron configurations Examples Chlorine: atomic number = 17, so 17 electrons to be placed in orbitals: 1 s 22 p 63 s 23 p 5 1 st shell 2 nd shell 3 rd shell add these to get total no. of electrons these electrons are higher in energy than 4 s, but nearer the nucleus Manganese: atomic number = 25: 1 s 22 p 63 s 23 p 64 s 13 d 5 4 s and 3 d orbitals 20 half full
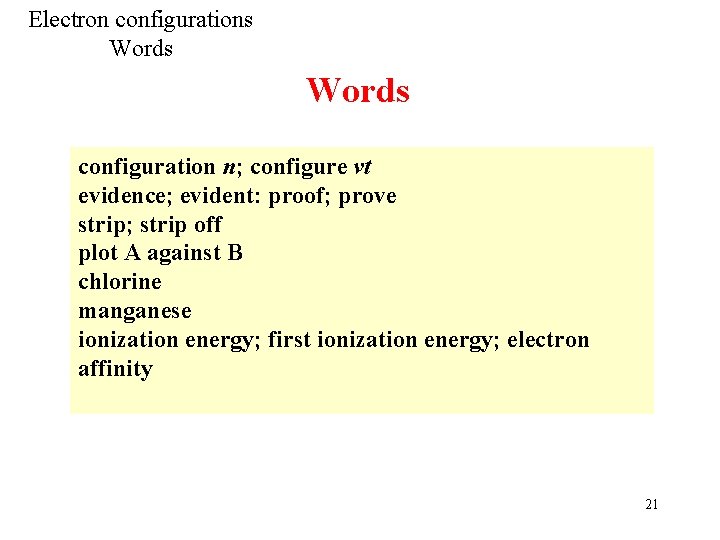
Electron configurations Words configuration n; configure vt evidence; evident: proof; prove strip; strip off plot A against B chlorine manganese ionization energy; first ionization energy; electron affinity 21
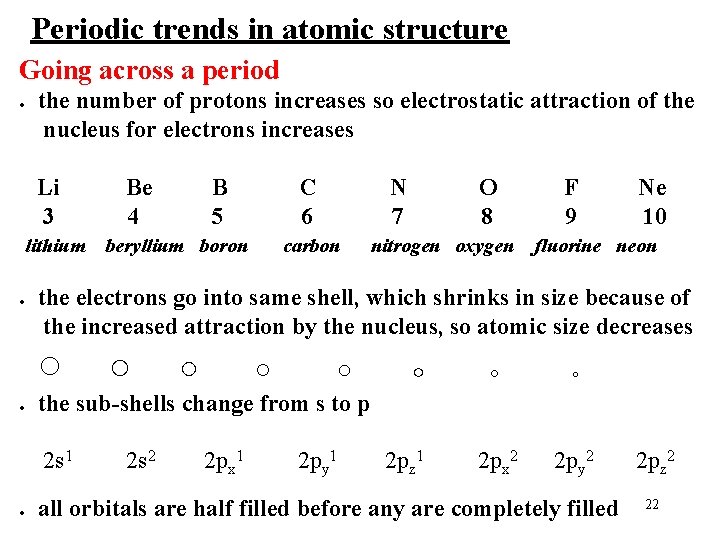
Periodic trends in atomic structure Going across a period ● the number of protons increases so electrostatic attraction of the nucleus for electrons increases Li 3 lithium ● C 6 beryllium boron carbon N 7 O 8 nitrogen oxygen ○ ○ the sub-shells change from s to p 2 s 1 ● B 5 F 9 Ne 10 fluorine neon the electrons go into same shell, which shrinks in size because of the increased attraction by the nucleus, so atomic size decreases ○ ● Be 4 2 s 2 2 px 1 2 py 1 ○ 2 pz 1 ○ ○ 2 px 2 2 py 2 all orbitals are half filled before any are completely filled 2 pz 2 22
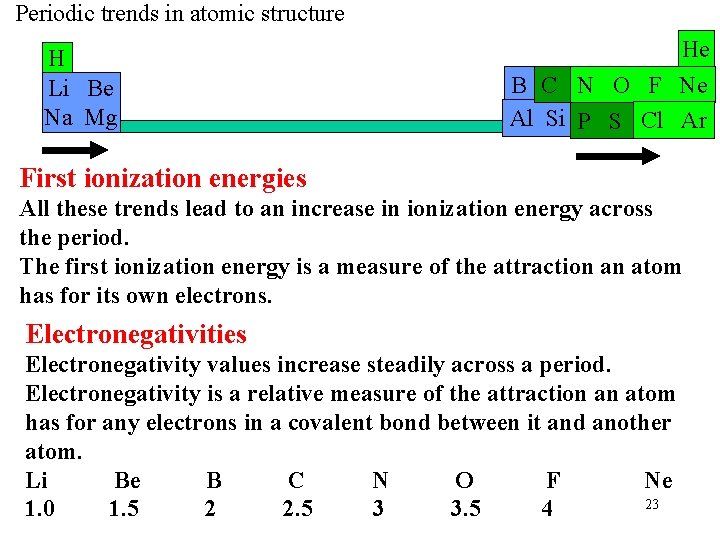
Periodic trends in atomic structure H Li Be Na Mg He B C N O F Ne Al Si P S Cl Ar First ionization energies All these trends lead to an increase in ionization energy across the period. The first ionization energy is a measure of the attraction an atom has for its own electrons. Electronegativities Electronegativity values increase steadily across a period. Electronegativity is a relative measure of the attraction an atom has for any electrons in a covalent bond between it and another atom. Li Be B C N O F Ne 23 1. 0 1. 5 2 2. 5 3 3. 5 4
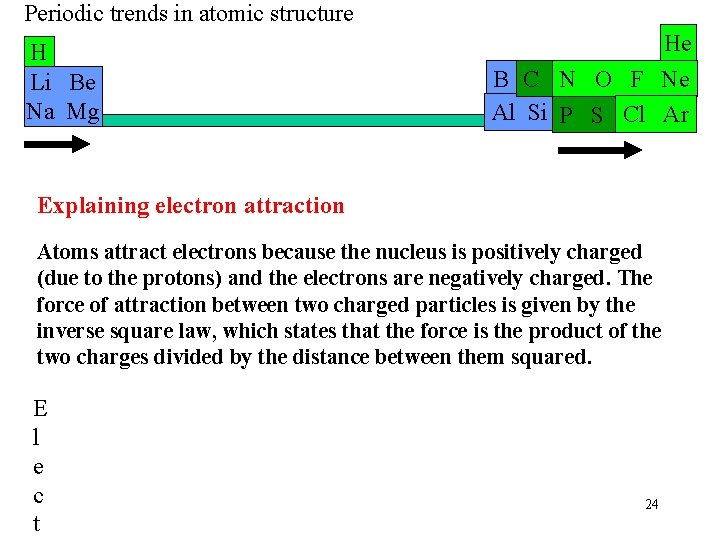
Periodic trends in atomic structure H Li Be Na Mg He B C N O F Ne Al Si P S Cl Ar Explaining electron attraction Atoms attract electrons because the nucleus is positively charged (due to the protons) and the electrons are negatively charged. The force of attraction between two charged particles is given by the inverse square law, which states that the force is the product of the two charges divided by the distance between them squared. E l e c t 24
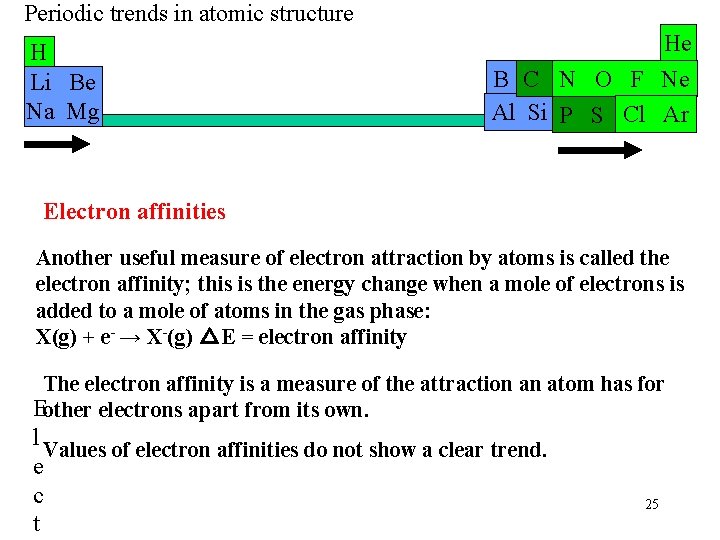
Periodic trends in atomic structure H Li Be Na Mg He B C N O F Ne Al Si P S Cl Ar Electron affinities Another useful measure of electron attraction by atoms is called the electron affinity; this is the energy change when a mole of electrons is added to a mole of atoms in the gas phase: X(g) + e- → X-(g) △E = electron affinity The electron affinity is a measure of the attraction an atom has for Eother electrons apart from its own. l Values of electron affinities do not show a clear trend. e c t 25
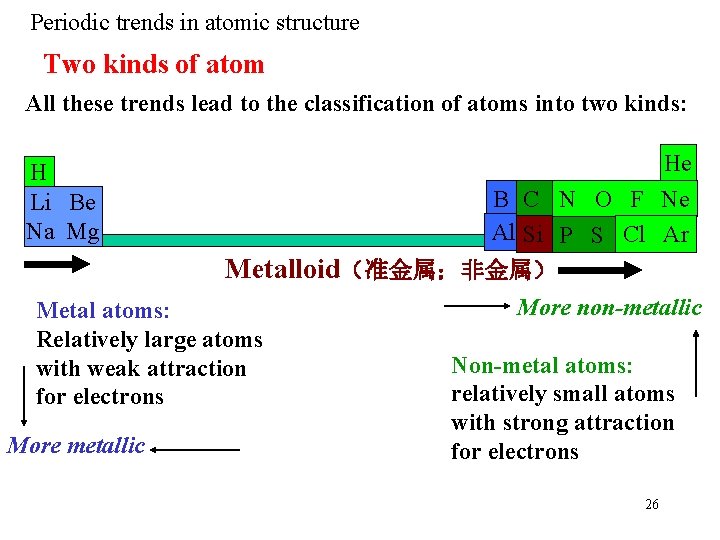
Periodic trends in atomic structure Two kinds of atom All these trends lead to the classification of atoms into two kinds: H Li Be Na Mg He B C N O F Ne Al Si P S Cl Ar Metalloid(准金属;非金属) Metal atoms: Relatively large atoms with weak attraction for electrons More metallic More non-metallic Non-metal atoms: relatively small atoms with strong attraction for electrons 26
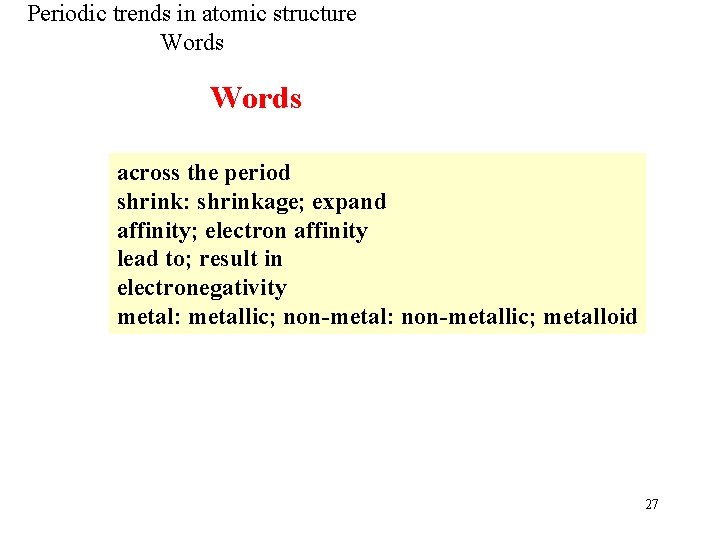
Periodic trends in atomic structure Words across the period shrink: shrinkage; expand affinity; electron affinity lead to; result in electronegativity metal: metallic; non-metal: non-metallic; metalloid 27
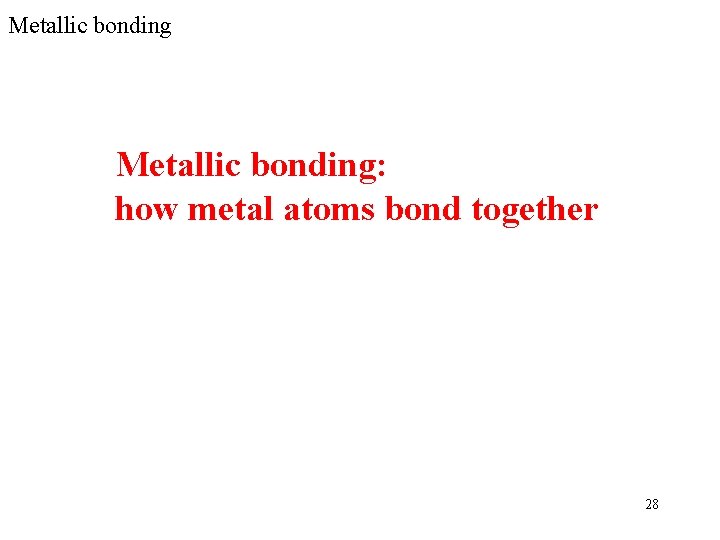
Metallic bonding: how metal atoms bond together 28
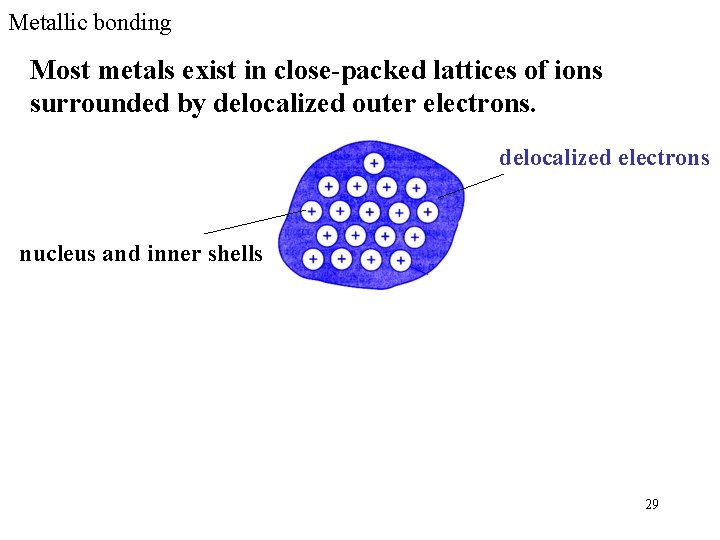
Metallic bonding Most metals exist in close-packed lattices of ions surrounded by delocalized outer electrons. delocalized electrons nucleus and inner shells 29
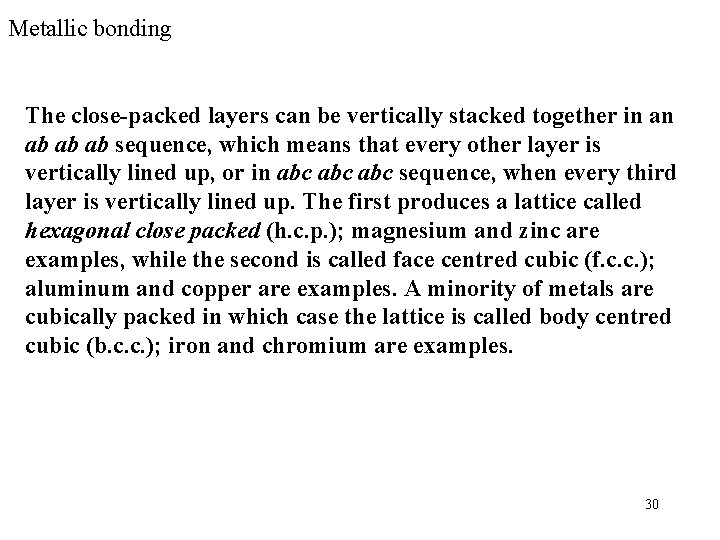
Metallic bonding The close-packed layers can be vertically stacked together in an ab ab ab sequence, which means that every other layer is vertically lined up, or in abc abc sequence, when every third layer is vertically lined up. The first produces a lattice called hexagonal close packed (h. c. p. ); magnesium and zinc are examples, while the second is called face centred cubic (f. c. c. ); aluminum and copper are examples. A minority of metals are cubically packed in which case the lattice is called body centred cubic (b. c. c. ); iron and chromium are examples. 30
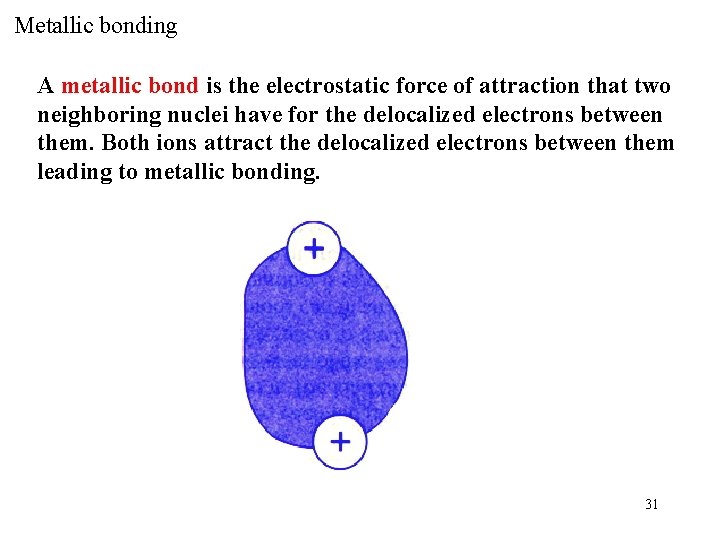
Metallic bonding A metallic bond is the electrostatic force of attraction that two neighboring nuclei have for the delocalized electrons between them. Both ions attract the delocalized electrons between them leading to metallic bonding. 31
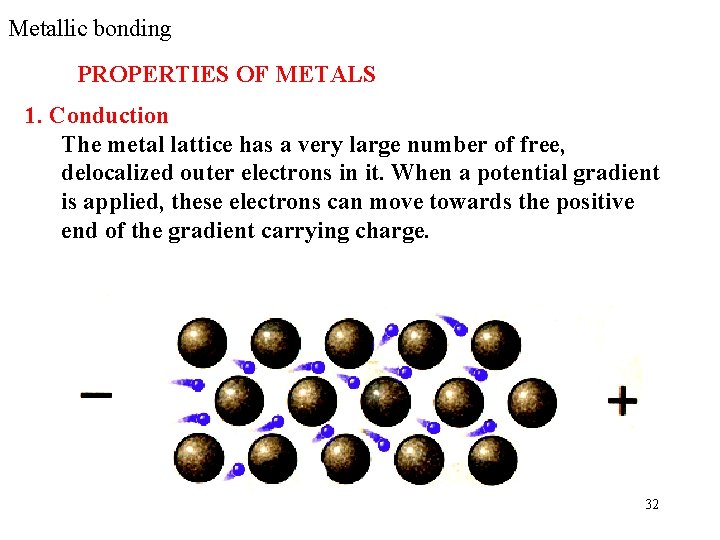
Metallic bonding PROPERTIES OF METALS 1. Conduction The metal lattice has a very large number of free, delocalized outer electrons in it. When a potential gradient is applied, these electrons can move towards the positive end of the gradient carrying charge. 32
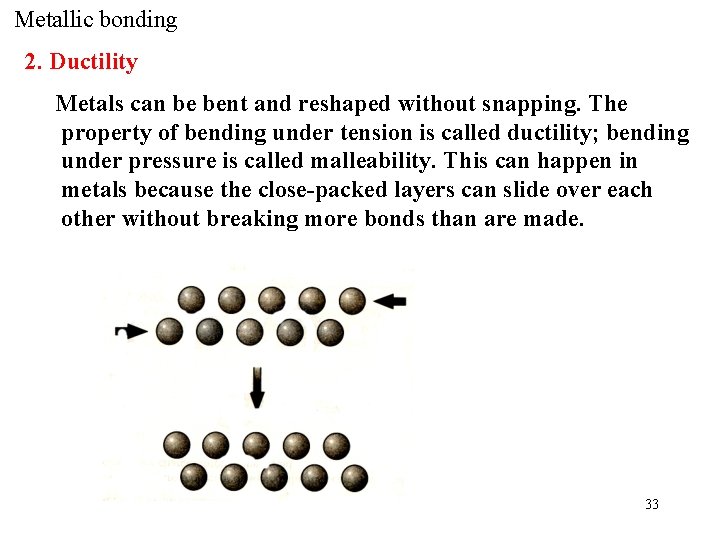
Metallic bonding 2. Ductility Metals can be bent and reshaped without snapping. The property of bending under tension is called ductility; bending under pressure is called malleability. This can happen in metals because the close-packed layers can slide over each other without breaking more bonds than are made. 33
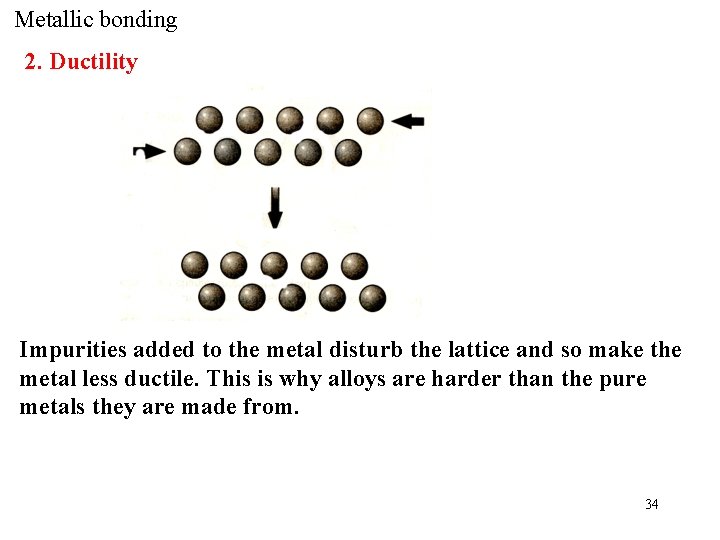
Metallic bonding 2. Ductility Impurities added to the metal disturb the lattice and so make the metal less ductile. This is why alloys are harder than the pure metals they are made from. 34
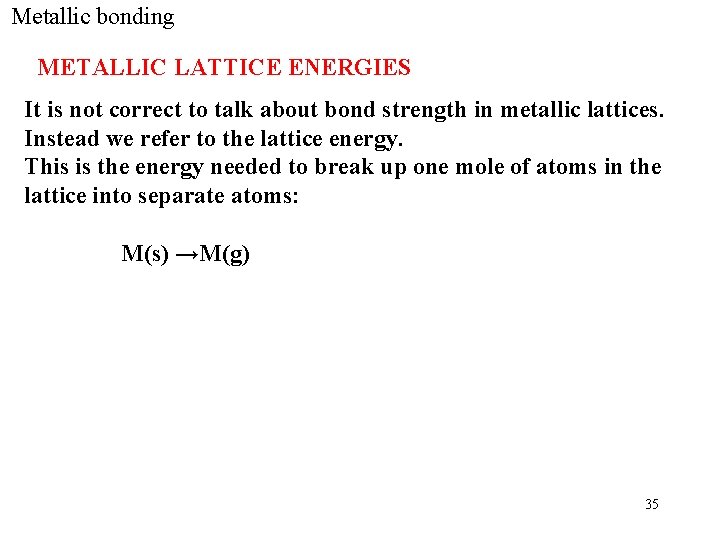
Metallic bonding METALLIC LATTICE ENERGIES It is not correct to talk about bond strength in metallic lattices. Instead we refer to the lattice energy. This is the energy needed to break up one mole of atoms in the lattice into separate atoms: M(s) →M(g) 35
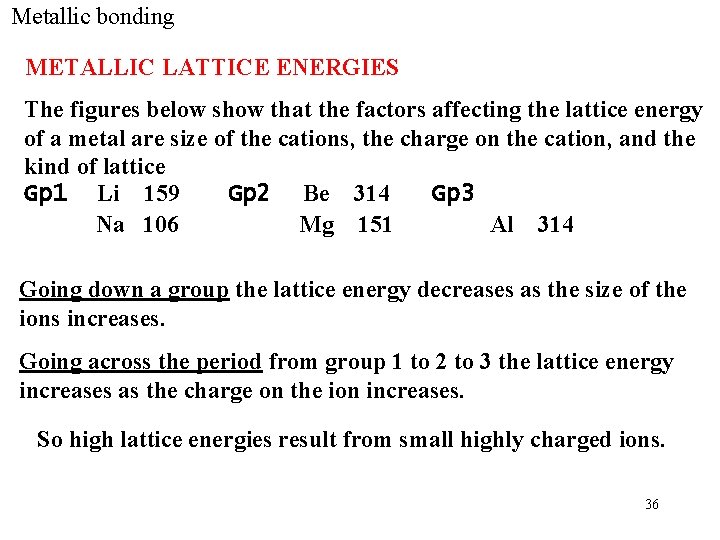
Metallic bonding METALLIC LATTICE ENERGIES The figures below show that the factors affecting the lattice energy of a metal are size of the cations, the charge on the cation, and the kind of lattice Gp 1 Li 159 Gp 2 Be 314 Gp 3 Na 106 Mg 151 Al 314 Going down a group the lattice energy decreases as the size of the ions increases. Going across the period from group 1 to 2 to 3 the lattice energy increases as the charge on the ion increases. So high lattice energies result from small highly charged ions. 36
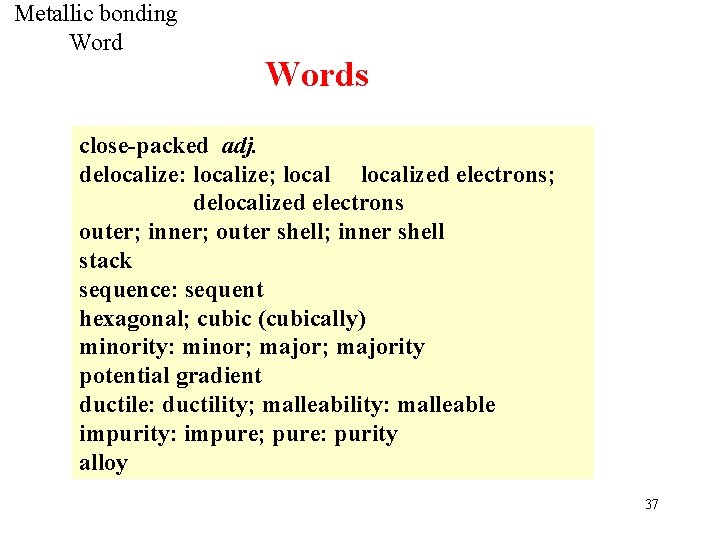
Metallic bonding Words close-packed adj. delocalize: localize; localized electrons; delocalized electrons outer; inner; outer shell; inner shell stack sequence: sequent hexagonal; cubic (cubically) minority: minor; majority potential gradient ductile: ductility; malleability: malleable impurity: impure; pure: purity alloy 37
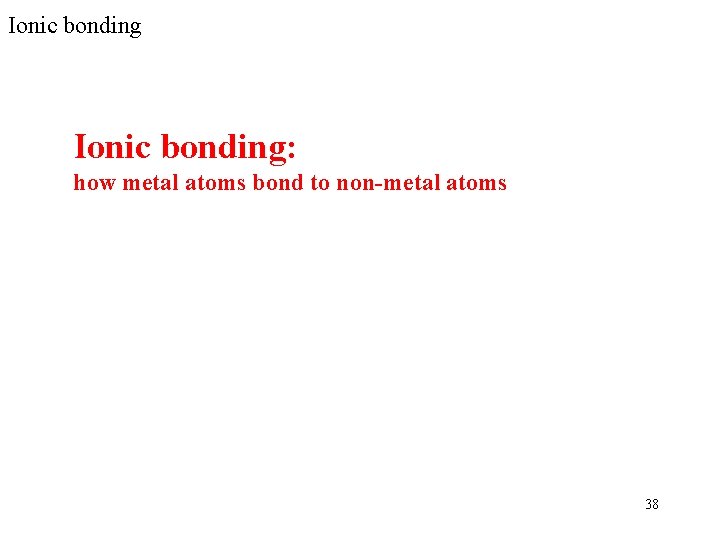
Ionic bonding: how metal atoms bond to non-metal atoms 38
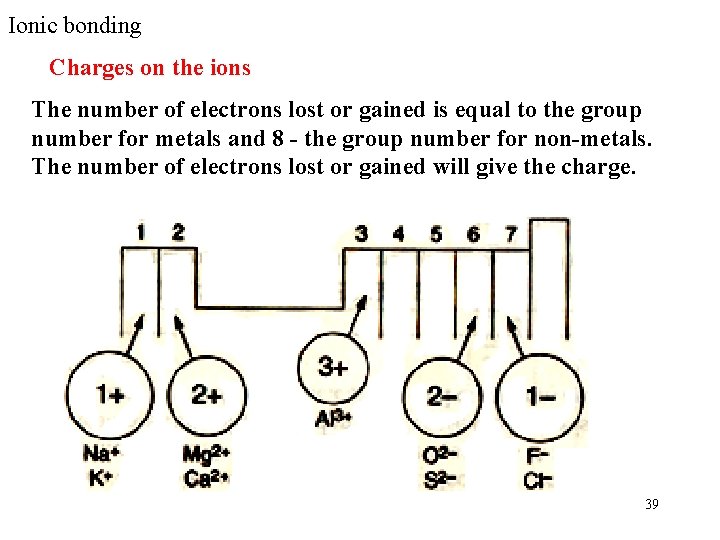
Ionic bonding Charges on the ions The number of electrons lost or gained is equal to the group number for metals and 8 - the group number for non-metals. The number of electrons lost or gained will give the charge. 39
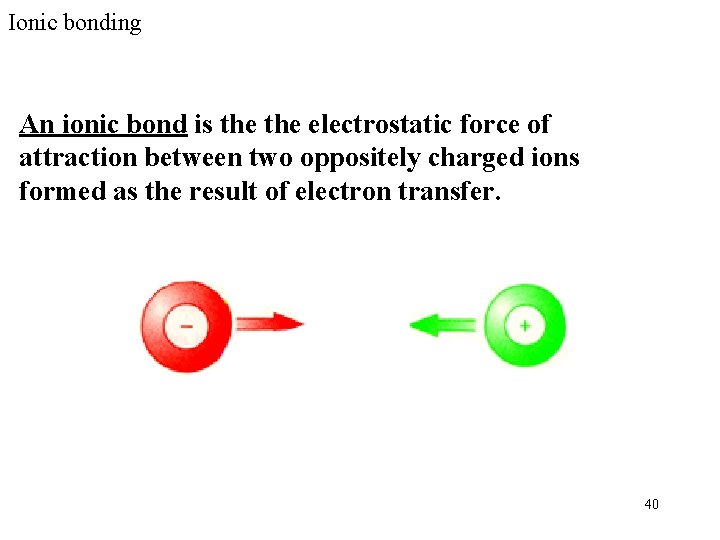
Ionic bonding An ionic bond is the electrostatic force of attraction between two oppositely charged ions formed as the result of electron transfer. 40
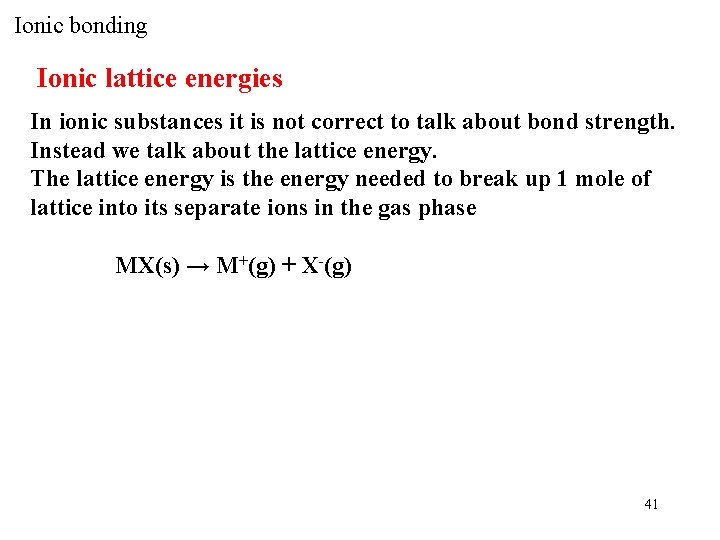
Ionic bonding Ionic lattice energies In ionic substances it is not correct to talk about bond strength. Instead we talk about the lattice energy. The lattice energy is the energy needed to break up 1 mole of lattice into its separate ions in the gas phase MX(s) → M+(g) + X-(g) 41
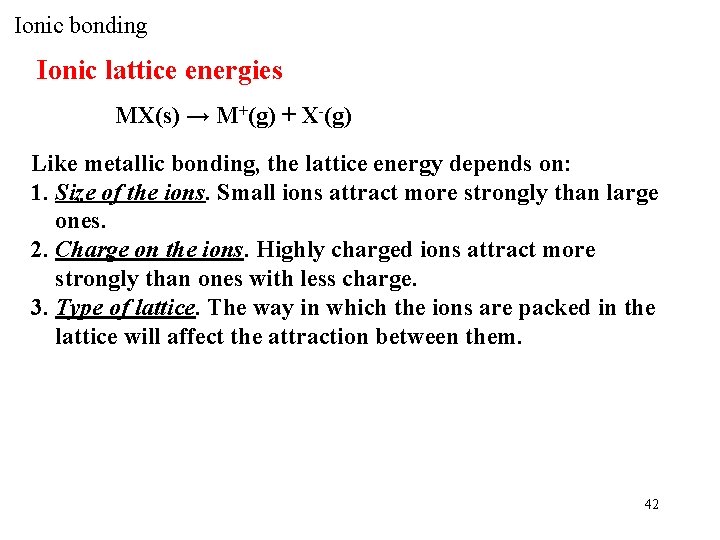
Ionic bonding Ionic lattice energies MX(s) → M+(g) + X-(g) Like metallic bonding, the lattice energy depends on: 1. Size of the ions. Small ions attract more strongly than large ones. 2. Charge on the ions. Highly charged ions attract more strongly than ones with less charge. 3. Type of lattice. The way in which the ions are packed in the lattice will affect the attraction between them. 42
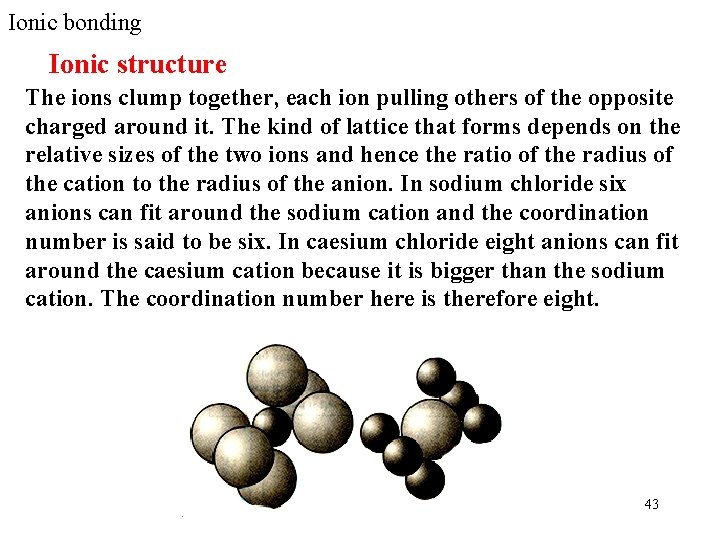
Ionic bonding Ionic structure The ions clump together, each ion pulling others of the opposite charged around it. The kind of lattice that forms depends on the relative sizes of the two ions and hence the ratio of the radius of the cation to the radius of the anion. In sodium chloride six anions can fit around the sodium cation and the coordination number is said to be six. In caesium chloride eight anions can fit around the caesium cation because it is bigger than the sodium cation. The coordination number here is therefore eight. 43
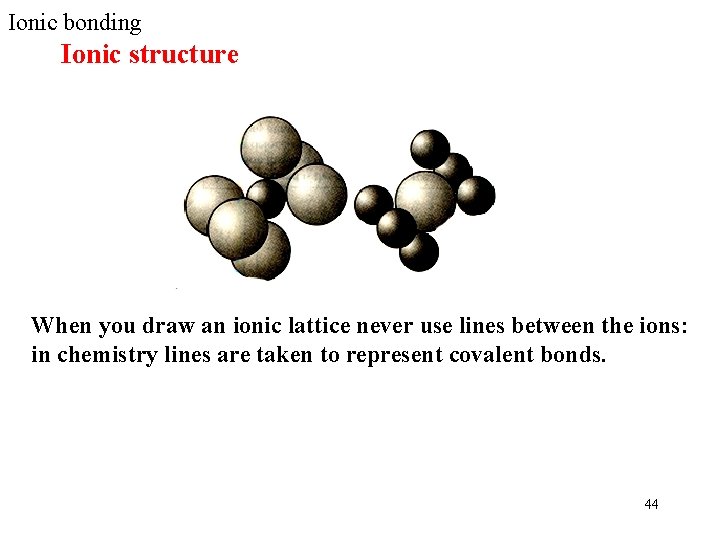
Ionic bonding Ionic structure When you draw an ionic lattice never use lines between the ions: in chemistry lines are taken to represent covalent bonds. 44
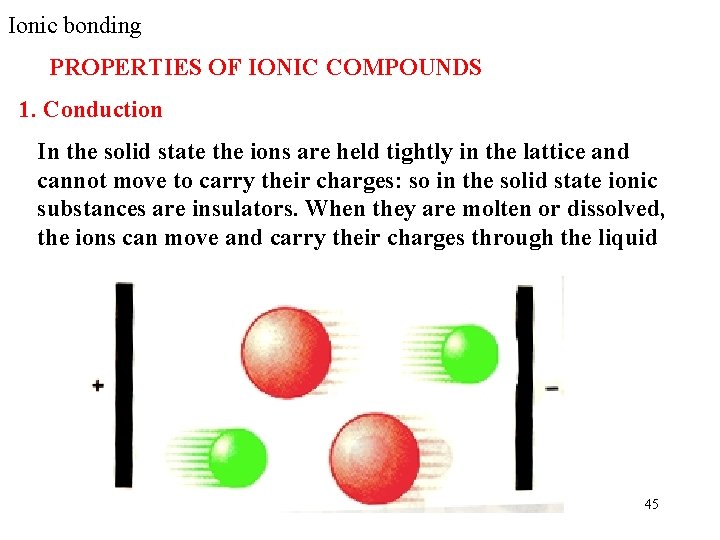
Ionic bonding PROPERTIES OF IONIC COMPOUNDS 1. Conduction In the solid state the ions are held tightly in the lattice and cannot move to carry their charges: so in the solid state ionic substances are insulators. When they are molten or dissolved, the ions can move and carry their charges through the liquid 45
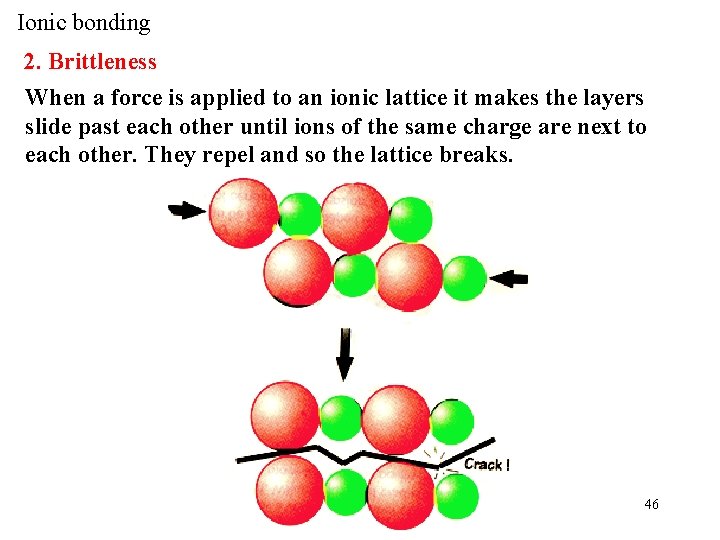
Ionic bonding 2. Brittleness When a force is applied to an ionic lattice it makes the layers slide past each other until ions of the same charge are next to each other. They repel and so the lattice breaks. 46
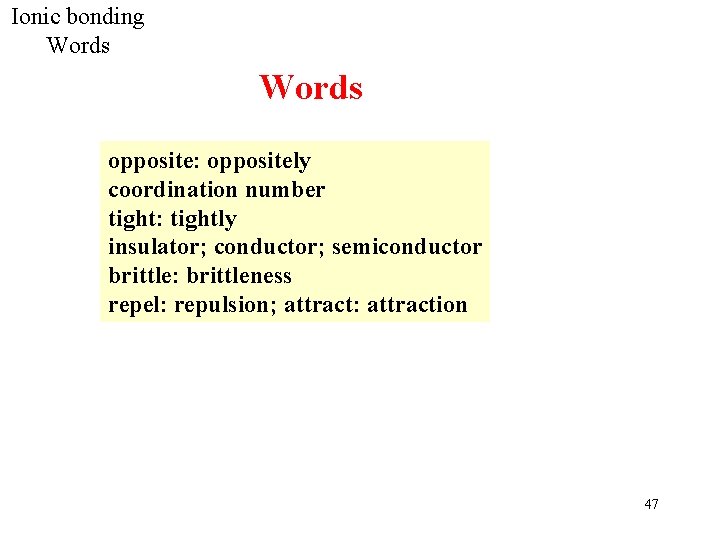
Ionic bonding Words opposite: oppositely coordination number tight: tightly insulator; conductor; semiconductor brittle: brittleness repel: repulsion; attract: attraction 47
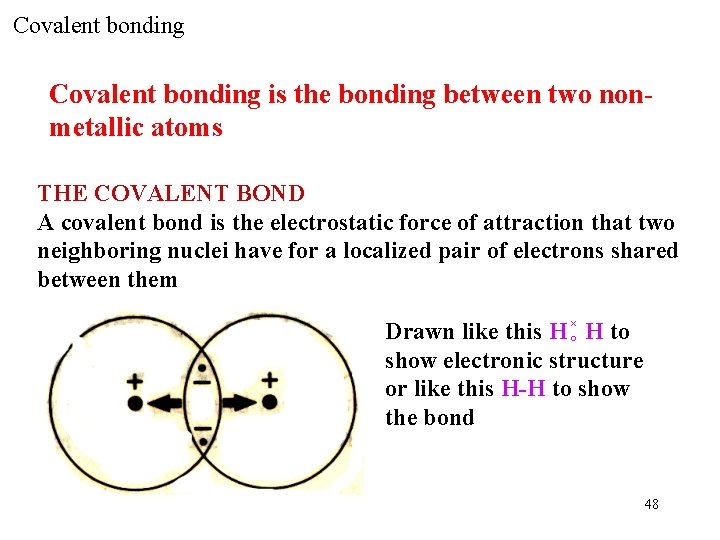
Covalent bonding is the bonding between two nonmetallic atoms THE COVALENT BOND A covalent bond is the electrostatic force of attraction that two neighboring nuclei have for a localized pair of electrons shared between them Drawn like this H ×○ H to show electronic structure or like this H-H to show the bond 48
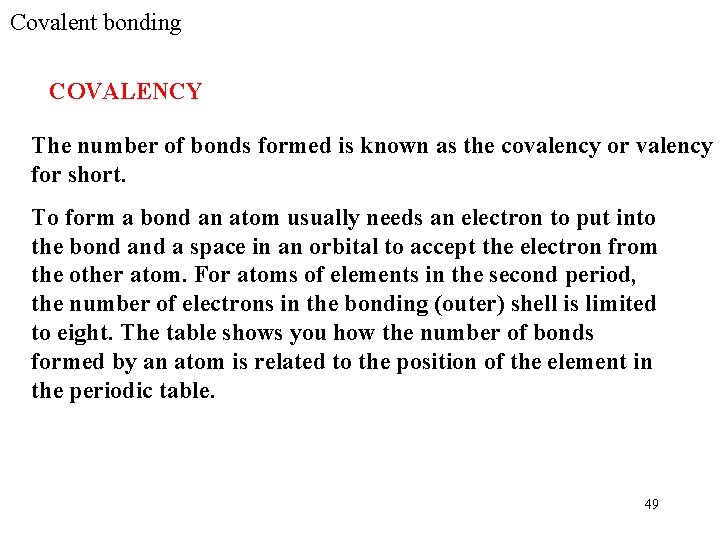
Covalent bonding COVALENCY The number of bonds formed is known as the covalency or valency for short. To form a bond an atom usually needs an electron to put into the bond a space in an orbital to accept the electron from the other atom. For atoms of elements in the second period, the number of electrons in the bonding (outer) shell is limited to eight. The table shows you how the number of bonds formed by an atom is related to the position of the element in the periodic table. 49
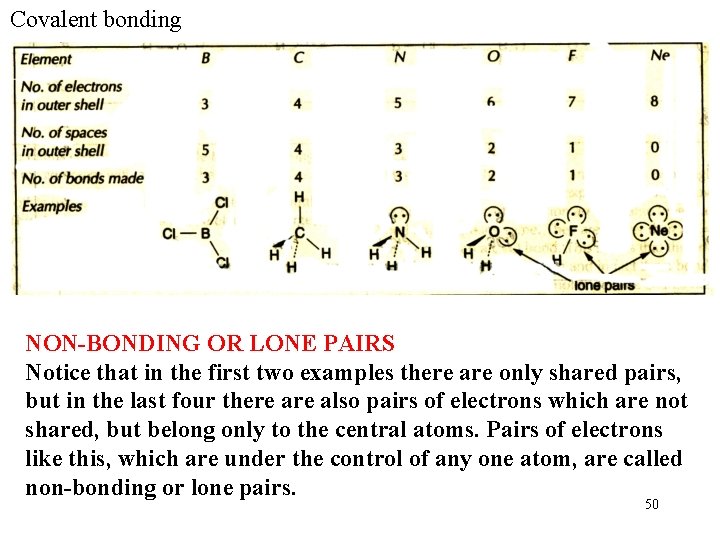
Covalent bonding NON-BONDING OR LONE PAIRS Notice that in the first two examples there are only shared pairs, but in the last four there also pairs of electrons which are not shared, but belong only to the central atoms. Pairs of electrons like this, which are under the control of any one atom, are called non-bonding or lone pairs. 50
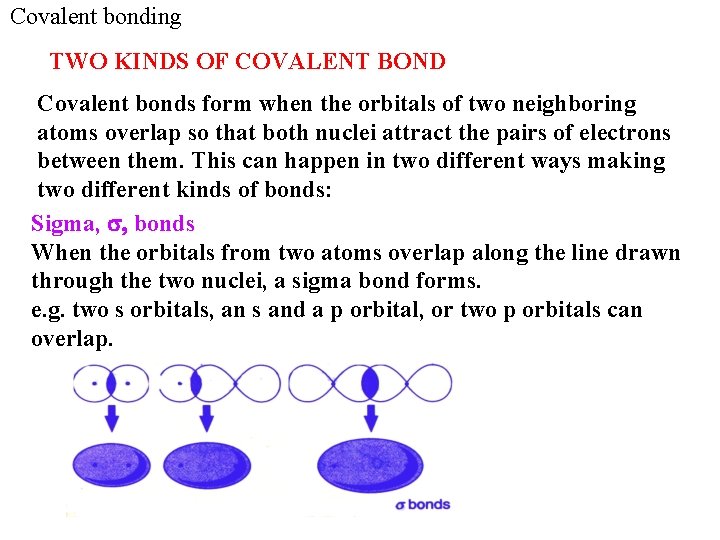
Covalent bonding TWO KINDS OF COVALENT BOND Covalent bonds form when the orbitals of two neighboring atoms overlap so that both nuclei attract the pairs of electrons between them. This can happen in two different ways making two different kinds of bonds: Sigma, s, bonds When the orbitals from two atoms overlap along the line drawn through the two nuclei, a sigma bond forms. e. g. two s orbitals, an s and a p orbital, or two p orbitals can overlap. 51
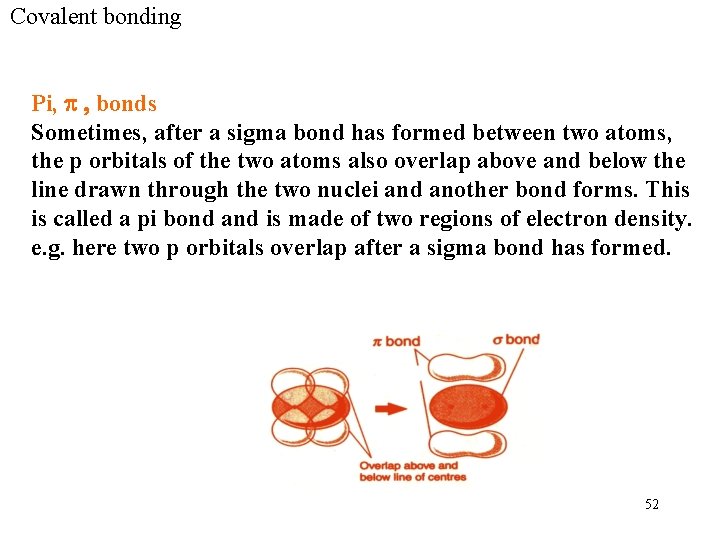
Covalent bonding Pi, p , bonds Sometimes, after a sigma bond has formed between two atoms, the p orbitals of the two atoms also overlap above and below the line drawn through the two nuclei and another bond forms. This is called a pi bond and is made of two regions of electron density. e. g. here two p orbitals overlap after a sigma bond has formed. 52
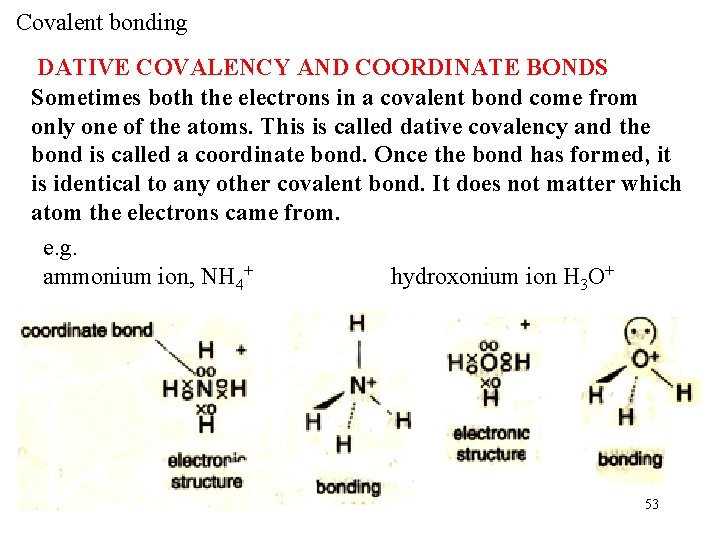
Covalent bonding DATIVE COVALENCY AND COORDINATE BONDS Sometimes both the electrons in a covalent bond come from only one of the atoms. This is called dative covalency and the bond is called a coordinate bond. Once the bond has formed, it is identical to any other covalent bond. It does not matter which atom the electrons came from. e. g. ammonium ion, NH 4+ hydroxonium ion H 3 O+ 53
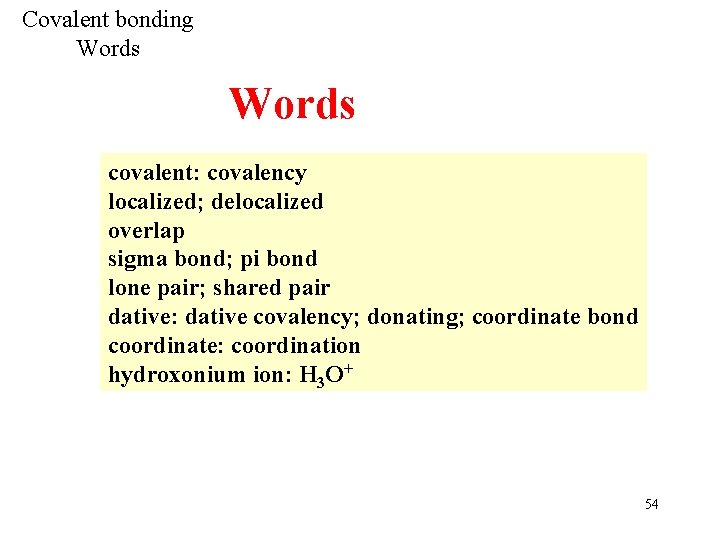
Covalent bonding Words covalent: covalency localized; delocalized overlap sigma bond; pi bond lone pair; shared pair dative: dative covalency; donating; coordinate bond coordinate: coordination hydroxonium ion: H 3 O+ 54
![Covalent bonding Quadruple bond K 2Re 2 Cl 8H 2 O Covalent bonding Quadruple bond K 2[Re 2 Cl 8]·H 2 O * * *](https://slidetodoc.com/presentation_image_h2/02c9dfa52b012cef7f984c73b1b96738/image-55.jpg)
Covalent bonding Quadruple bond K 2[Re 2 Cl 8]·H 2 O * * * Quintuple bond 55
Two protons in an atomic nucleus are typically
Protons and neutrons size
Find the number of neutrons au
Mass number of lithium
Sulfur number of neutrons protons and electrons
Element scavenger hunt answers
12c6 number of protons and neutrons
Ytterbium number of protons
Basic atomic structure worksheet
Can an atom have more neutrons than protons
Mass of protons neutrons electrons
Can an atom have more neutrons than protons
Which number is protons on periodic table
Lithium protons neutrons electrons
Can an atom have more neutrons than protons
Chromium 58 neutrons
400 bc democritus
39k+ protons neutrons electrons
39k+ protons neutrons electrons
U 238 protons neutrons electrons
Os atomos sao formados por protons neutrons e eletrons
Nitrogen
Which atom contains exactly 15 protons
Nonmetal period 3 mass 32
Atomic number of o
Electric forces within an atomic nucleus tend to
Relative atomic mass of beryllium
Is atomic mass and relative atomic mass the same
Atomic mass and atomic number difference
Periodic table tends
Atomic raduis
Atomic number vs atomic radius
Glycerophospholipid structure
Structure of a nucleus
Atomic structure and properties ap chemistry
Ap chemistry atomic structure and periodicity
Structure of the atom and the periodic table
Periodic table ape man
Ap chemistry chapter 7 atomic structure and periodicity
What is interatomic bonding
Chapter 7 atomic structure and periodicity
Element name
The indicated structure contains
Which sentence contains parallel structure?
Subatomic particles symbol
Http //education.jlab.org/qa/pen number.html
Lithium ion number of neutrons
Atomic structure jeopardy
Neutrons in hydrogen
Sonda de neutrons
Colbot-60 has how many neutrons?
Colbot-60 has how many neutrons?
How many neutrons does silicon 29 have
Atoms
Number of neutrons helium
How are neutron stars formed