SACE Stage 2 Physics The Structure of The
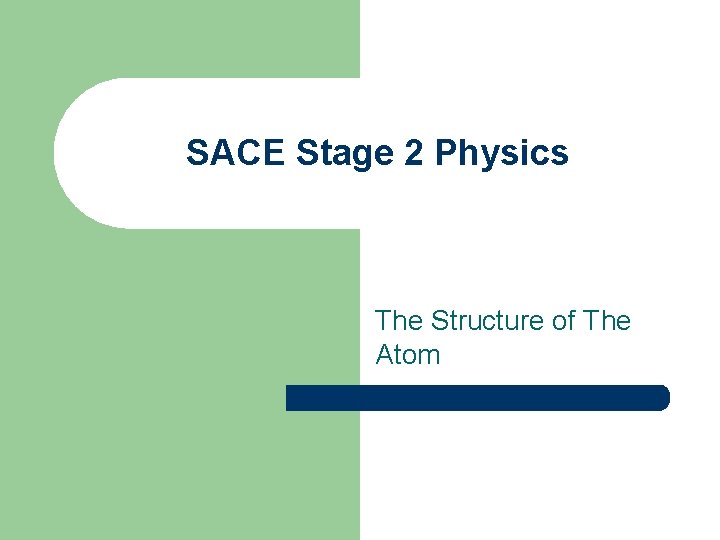
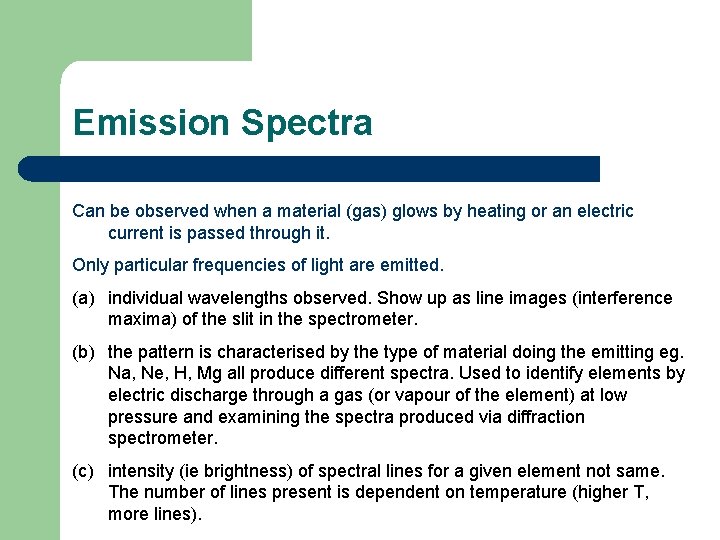
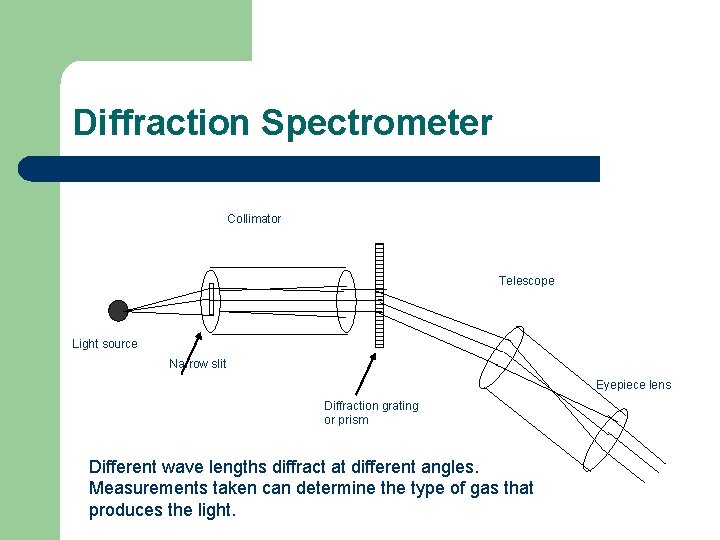
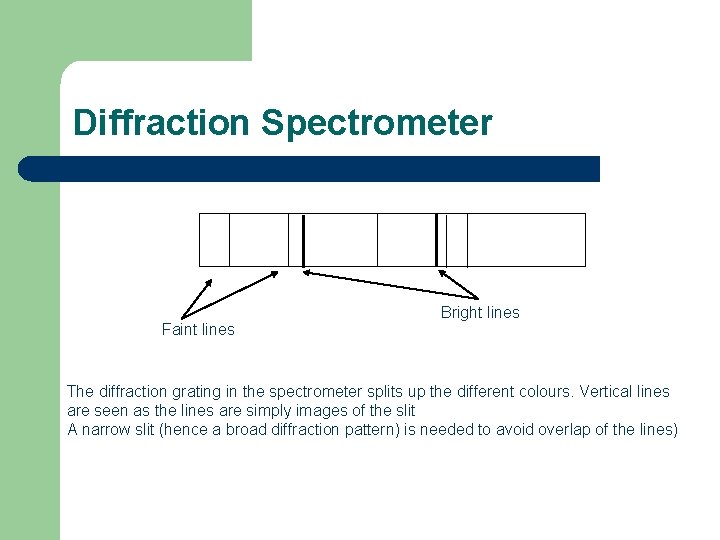
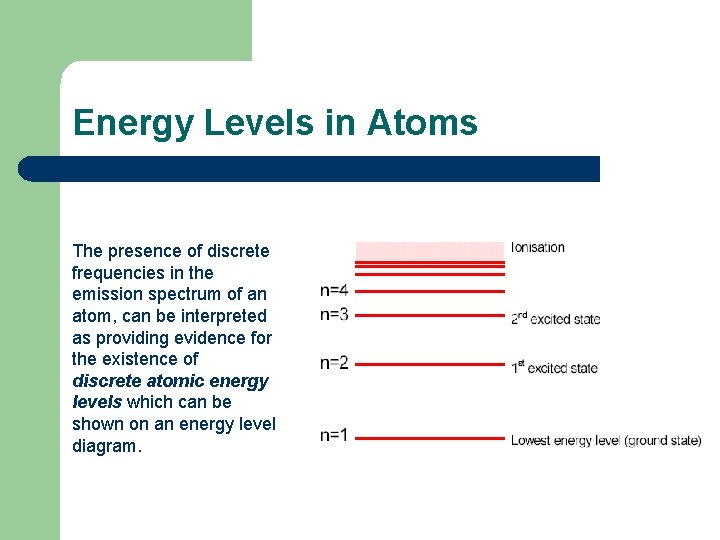
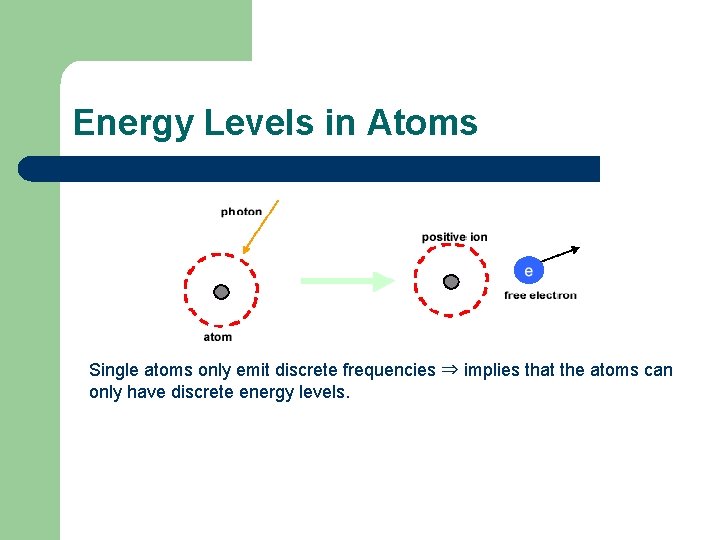
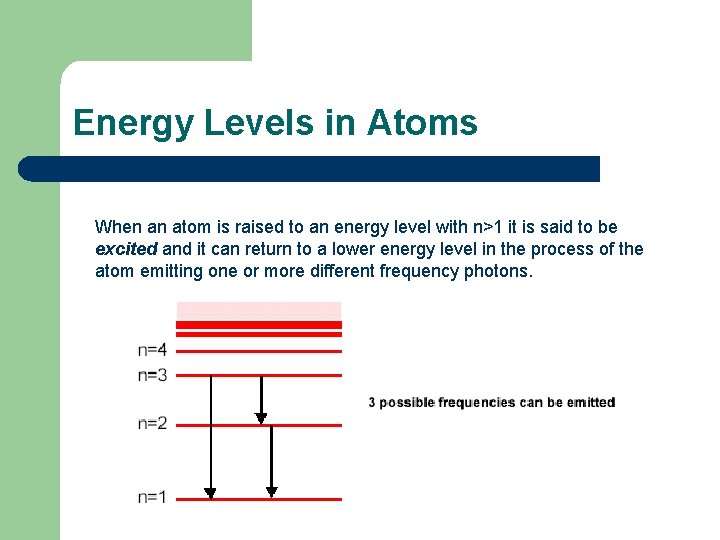
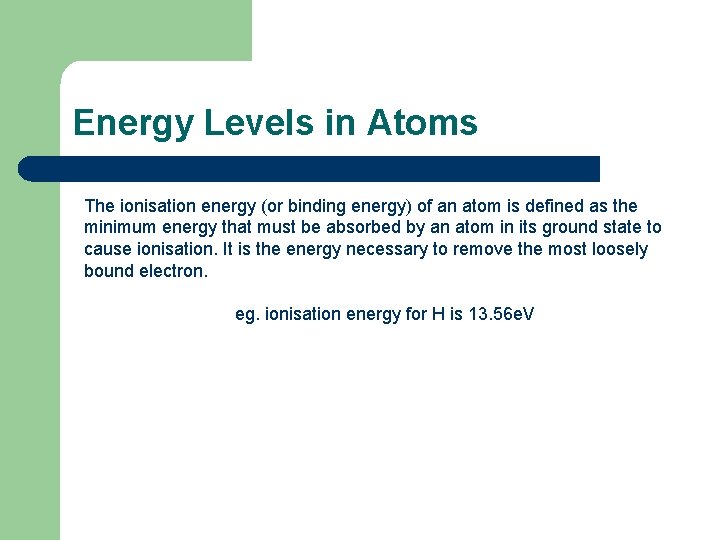
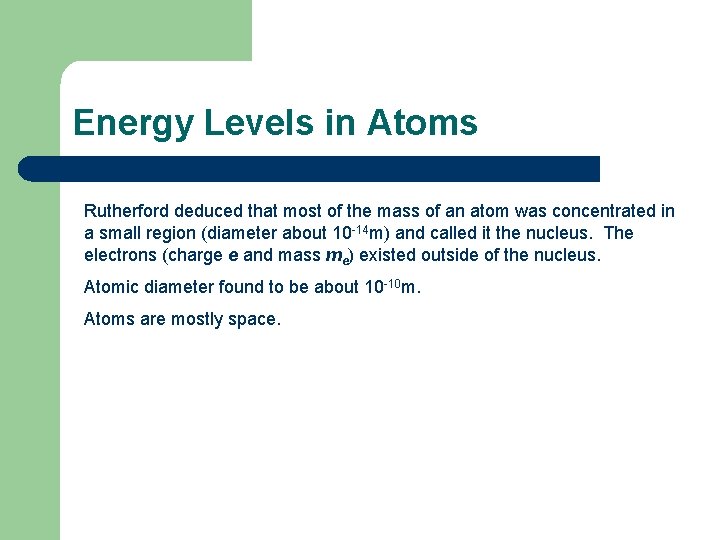
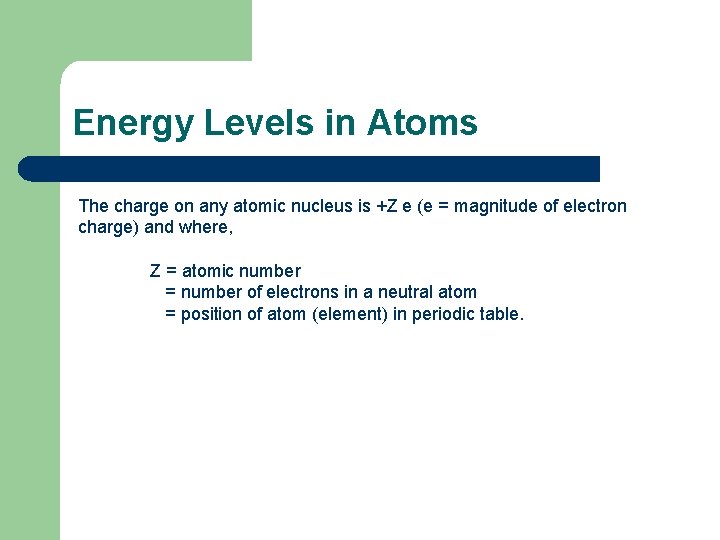
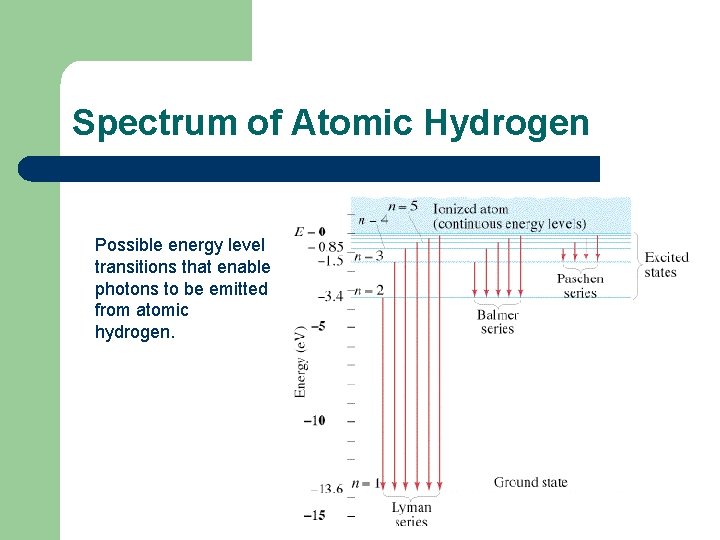
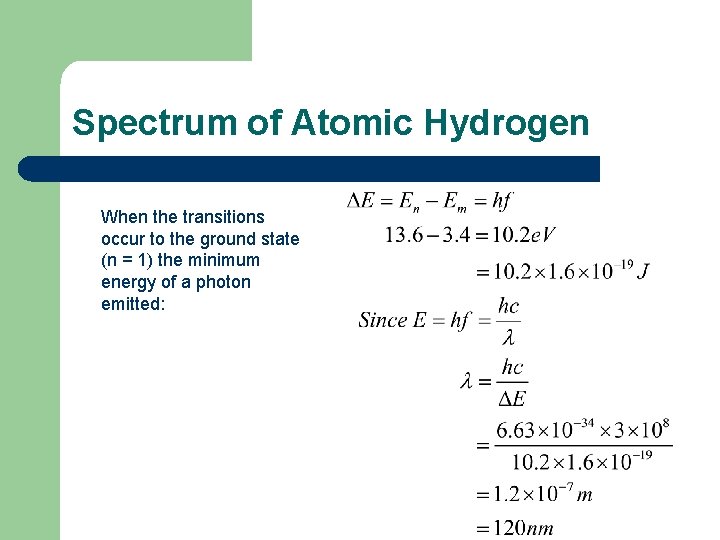
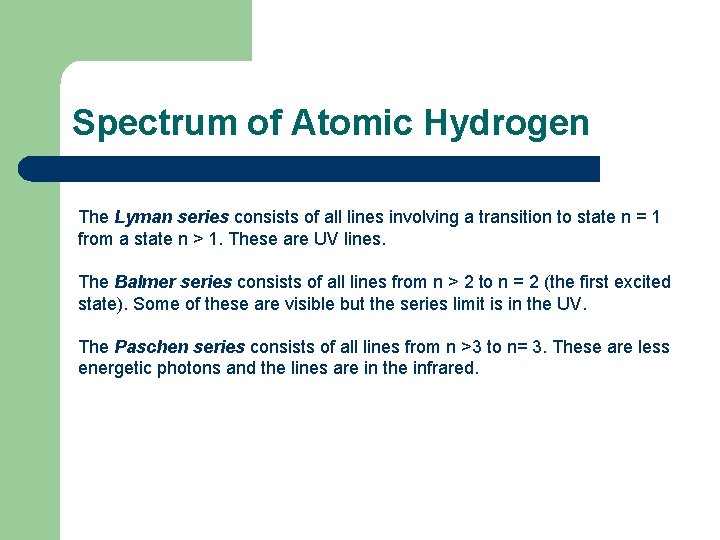
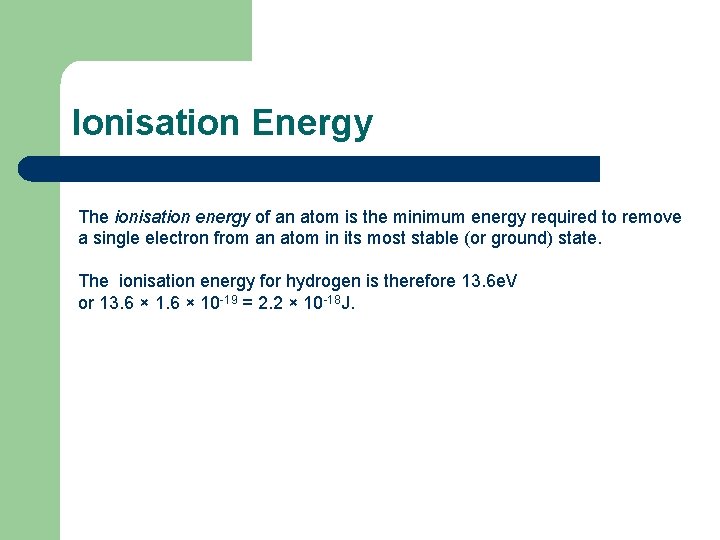
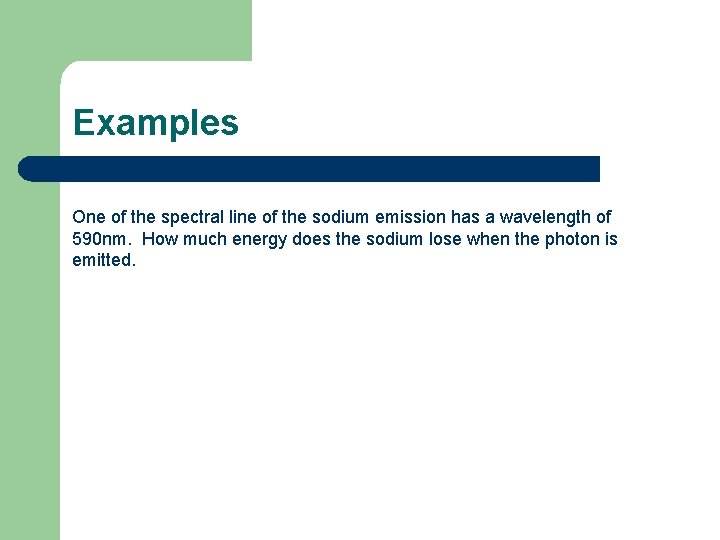
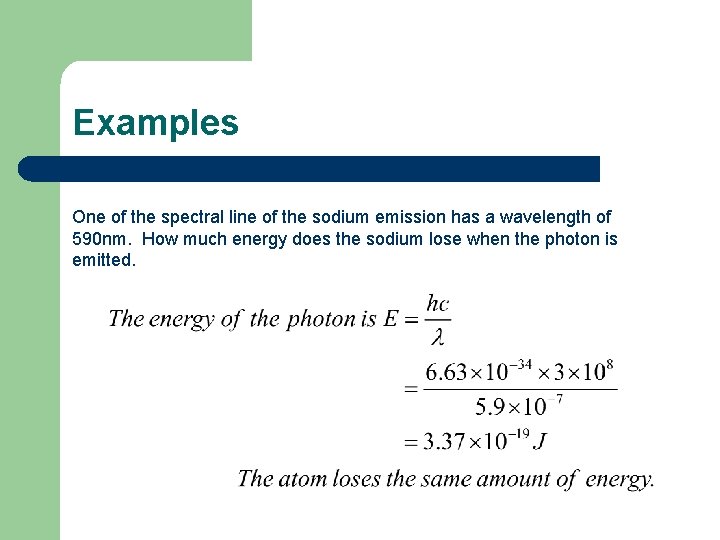
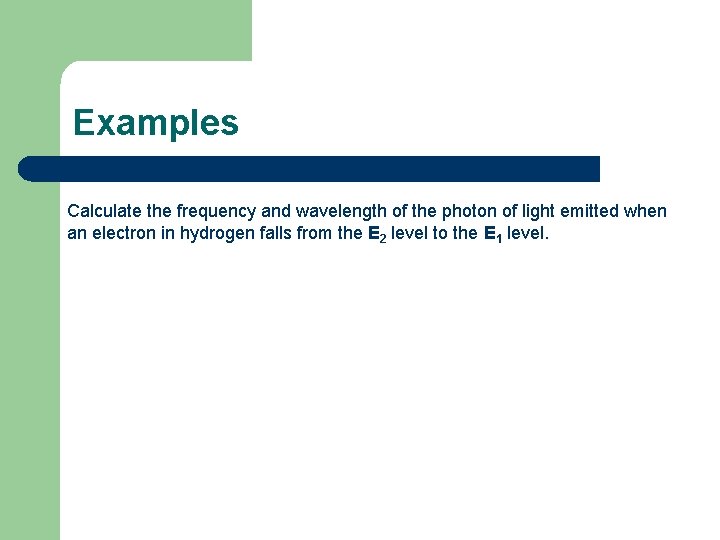
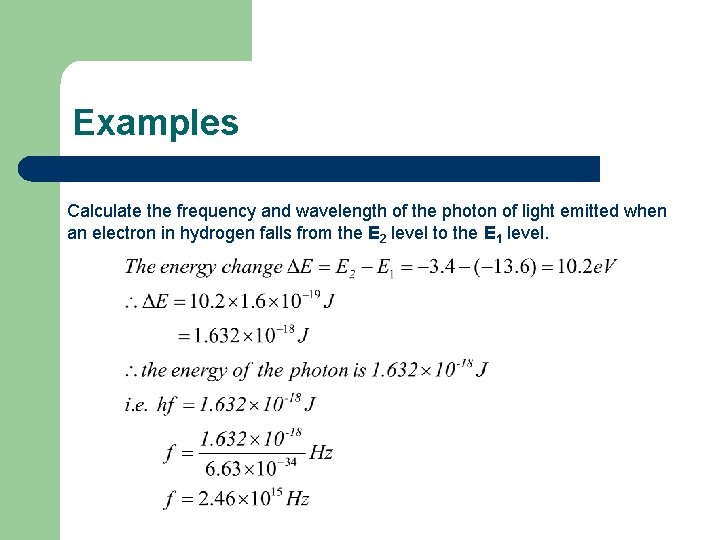
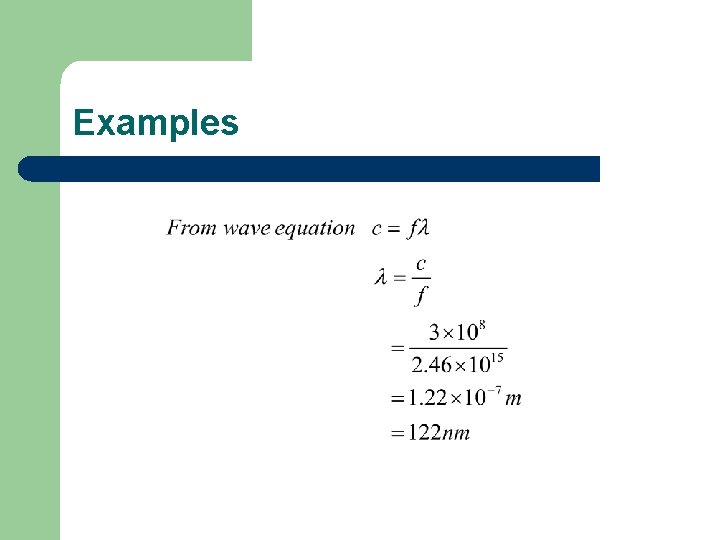
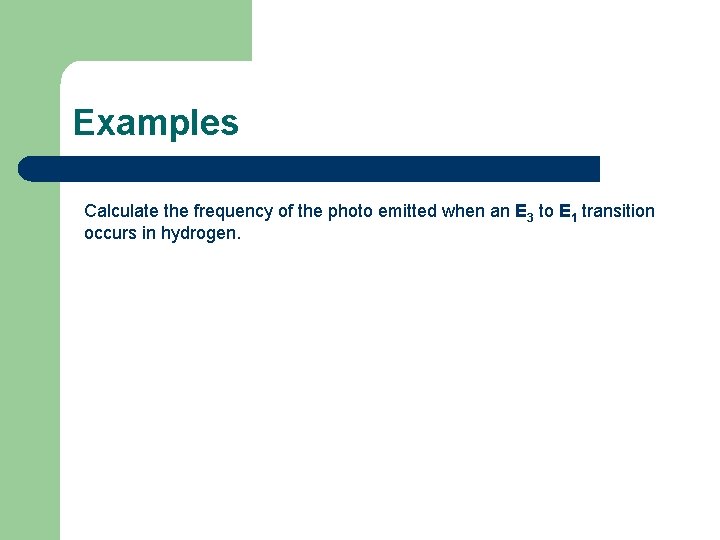
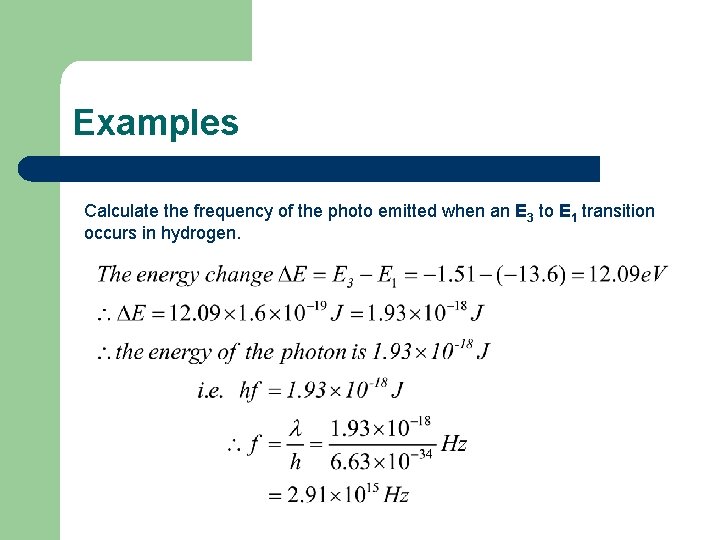
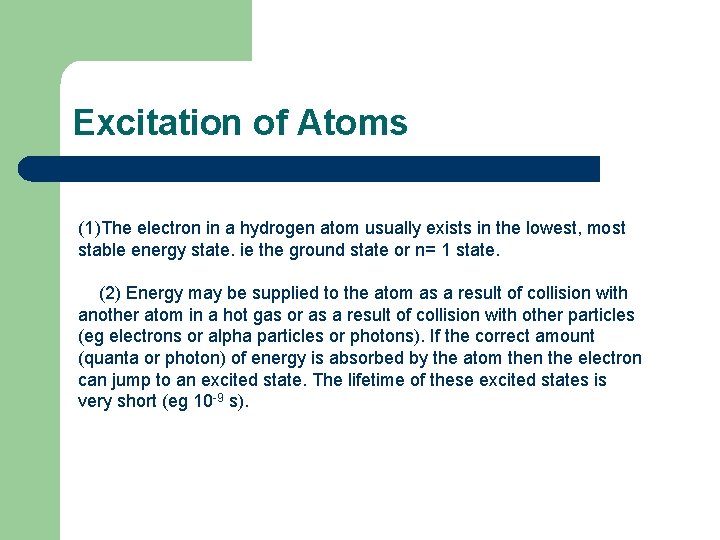
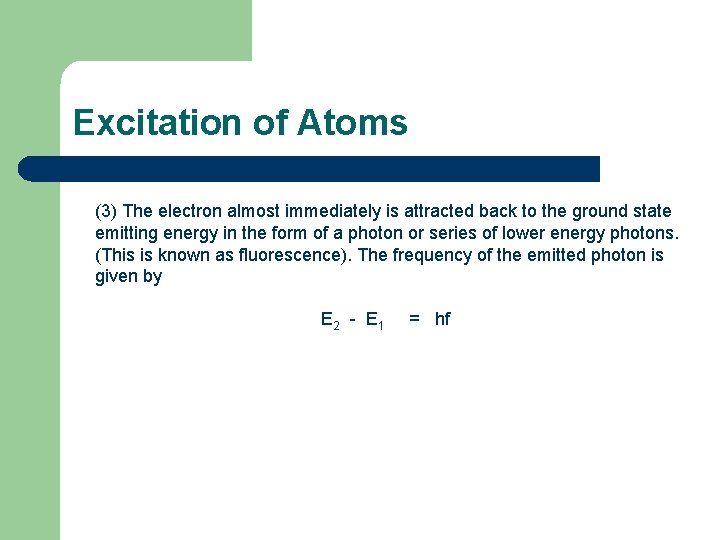
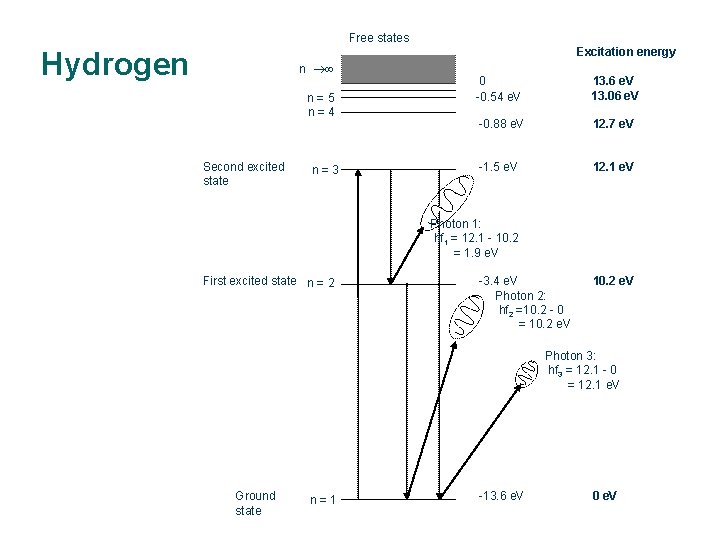
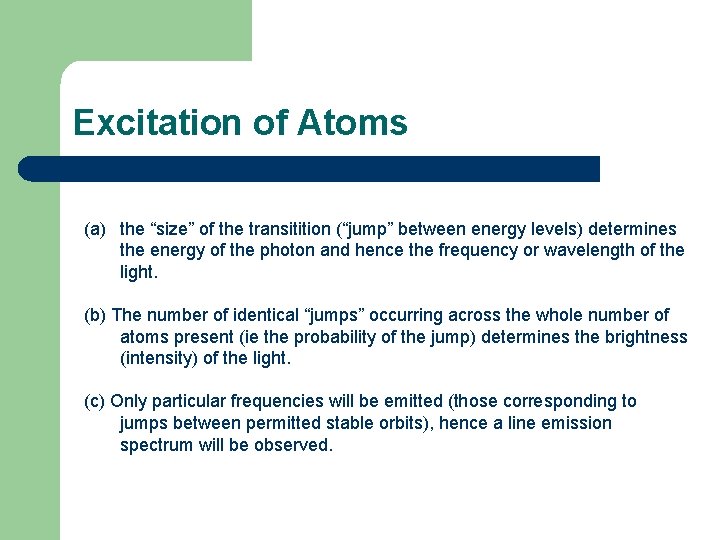
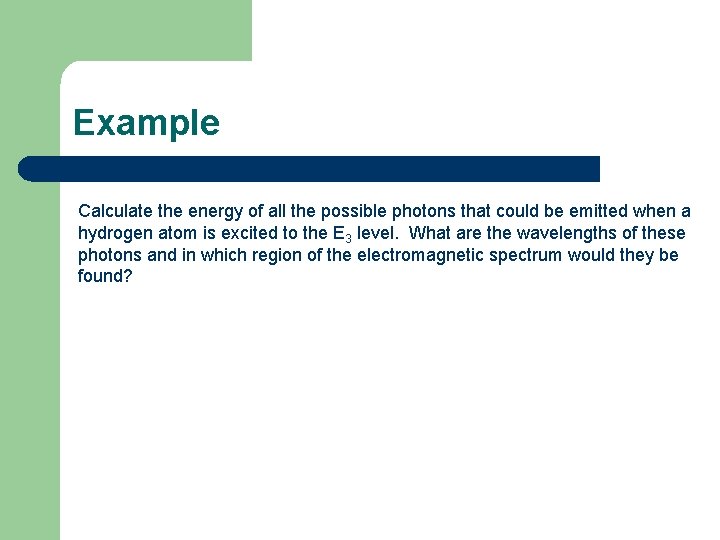
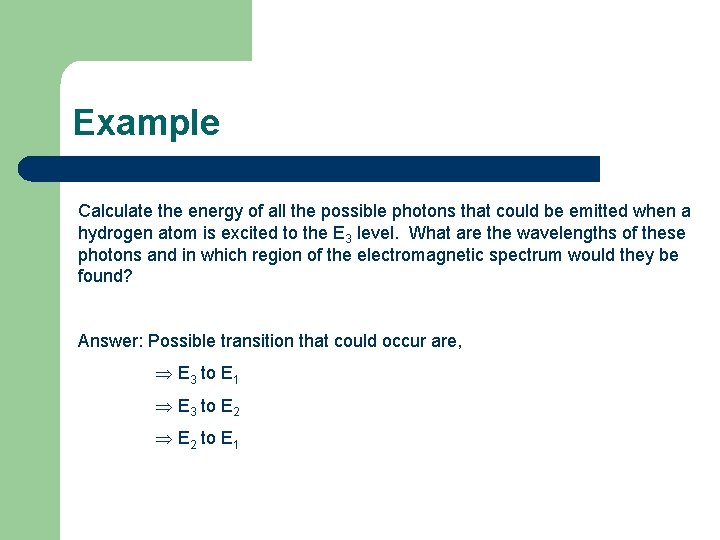
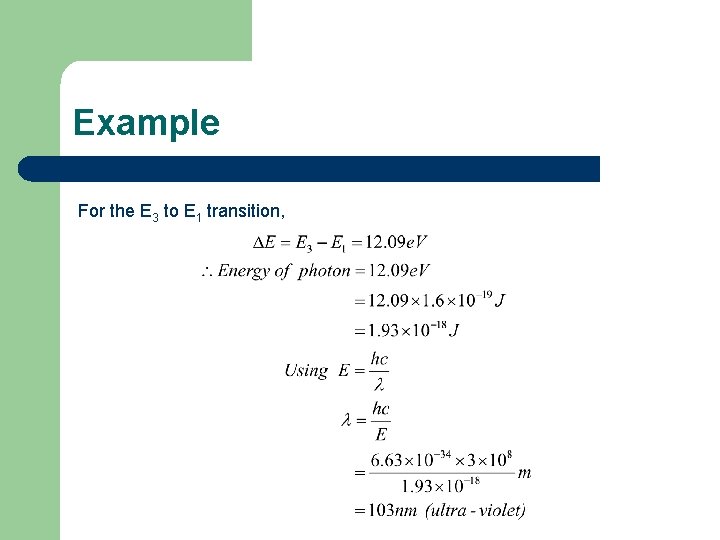
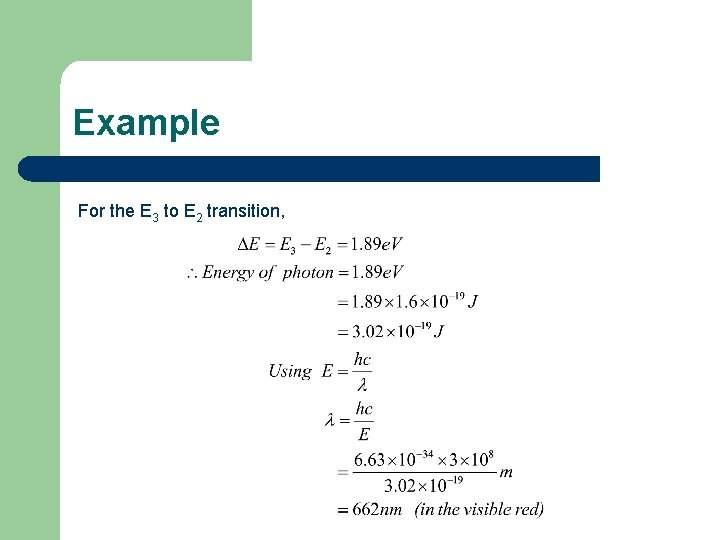
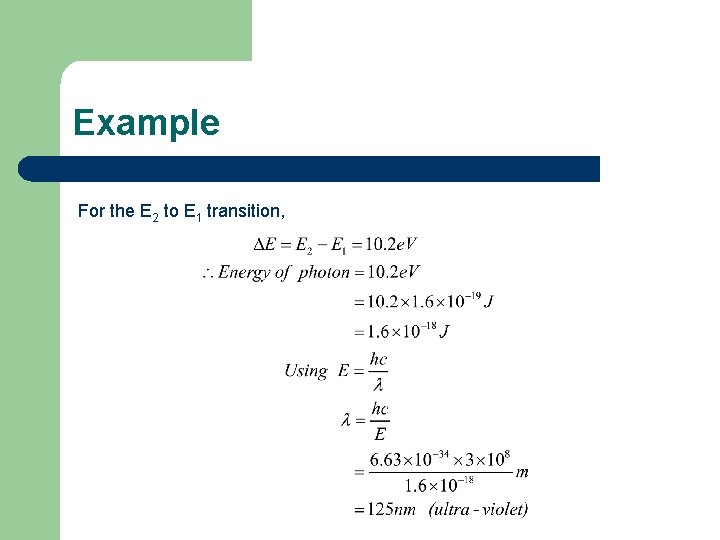
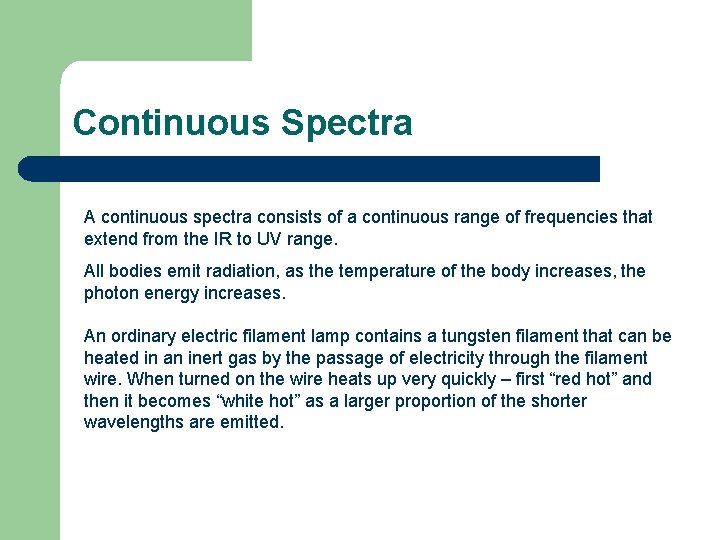
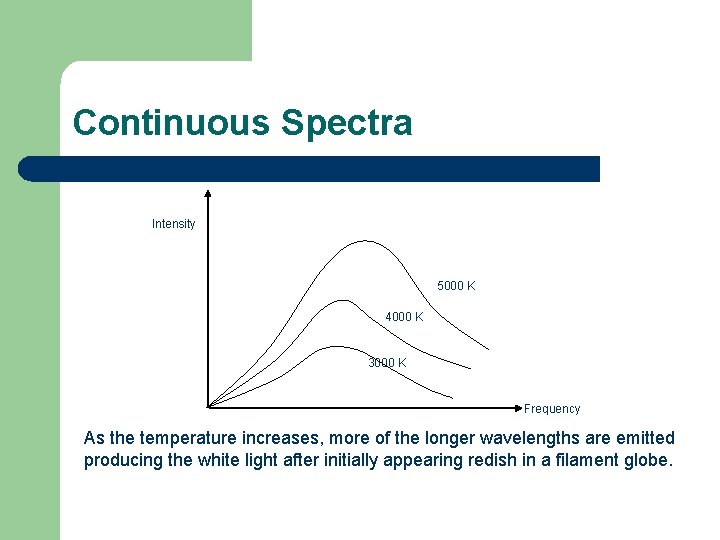
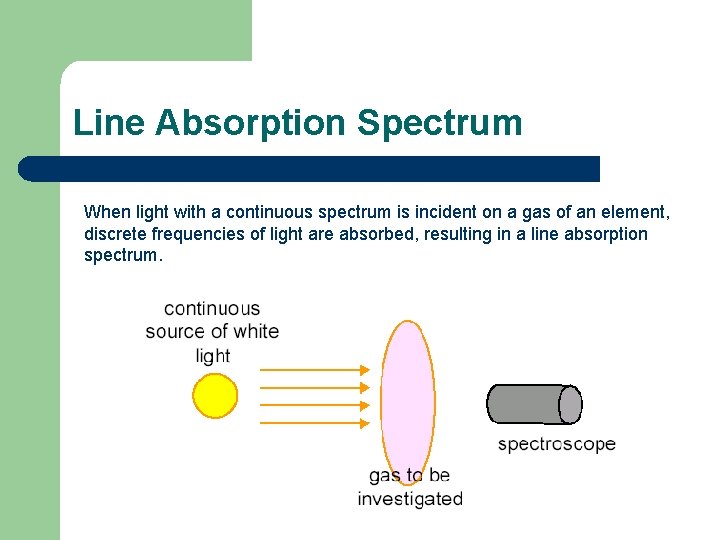
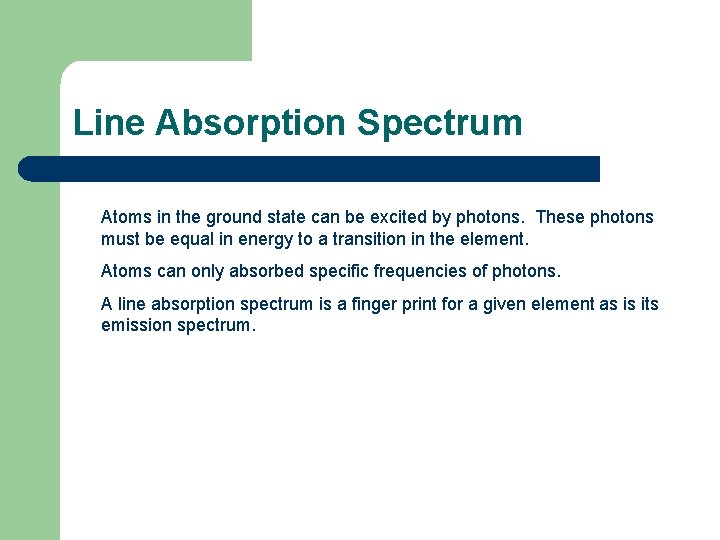
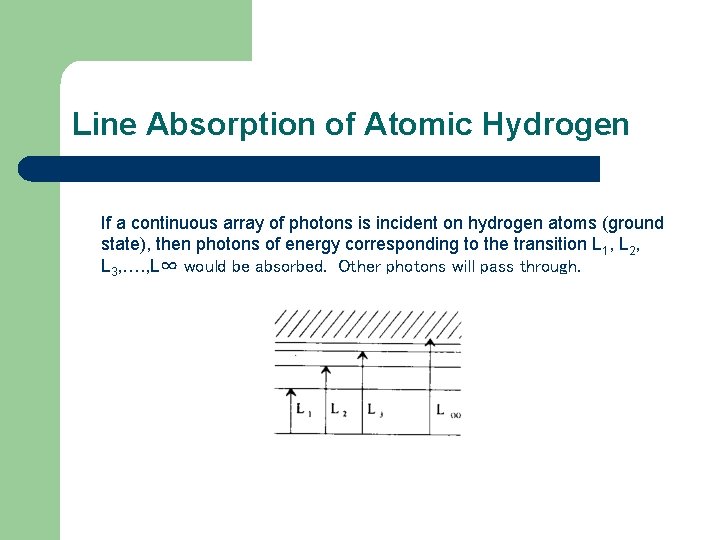
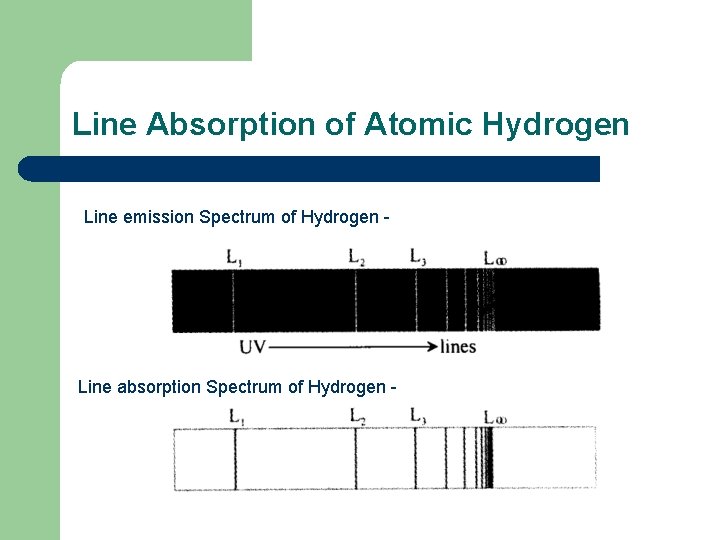
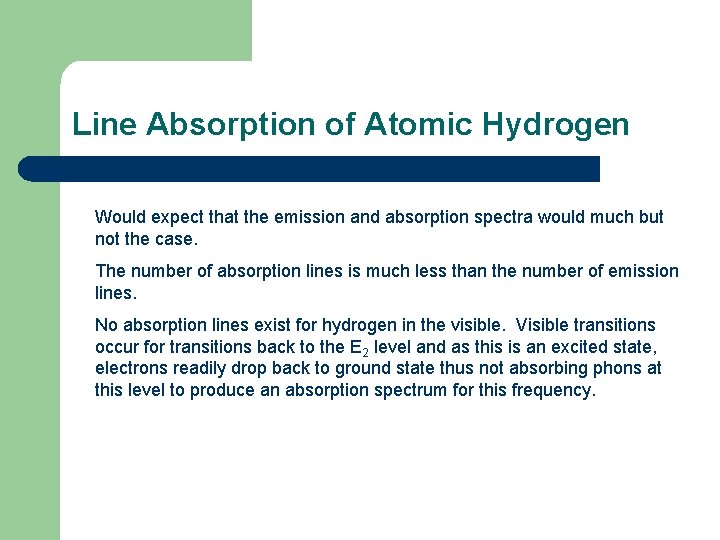
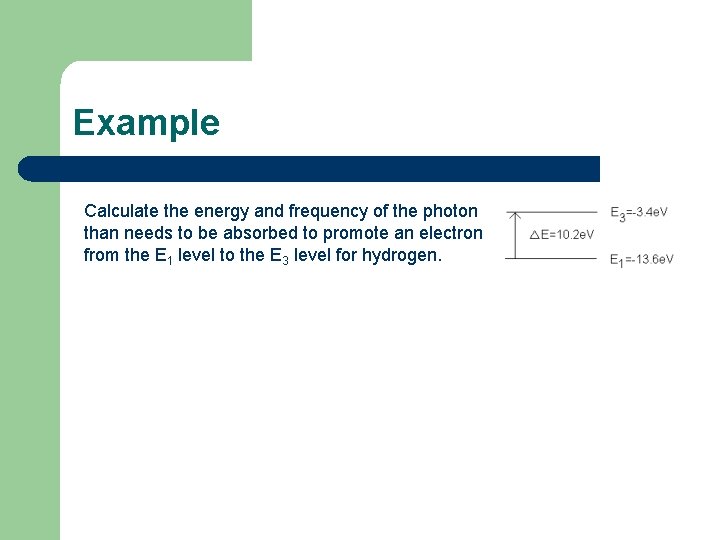
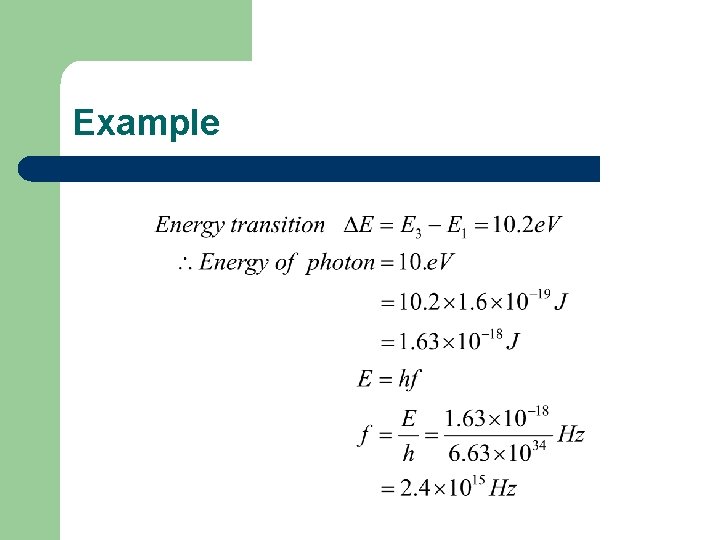
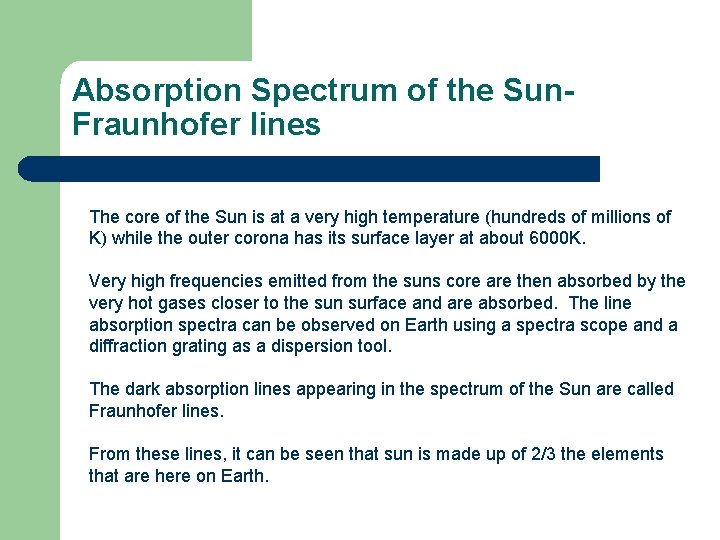
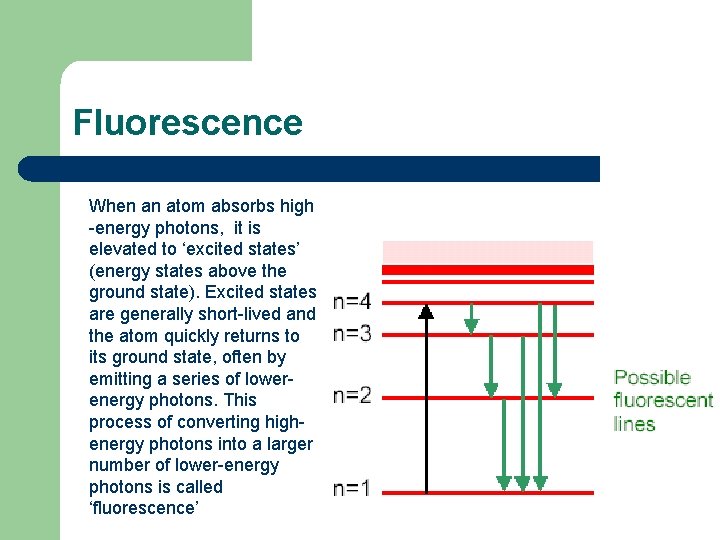
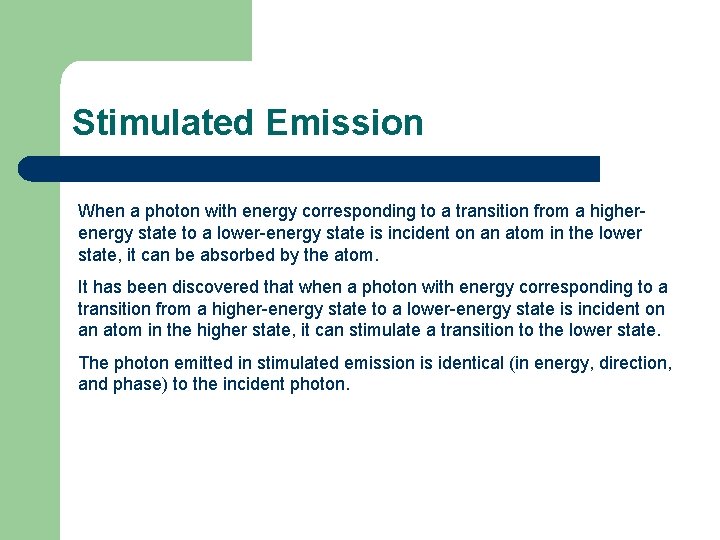
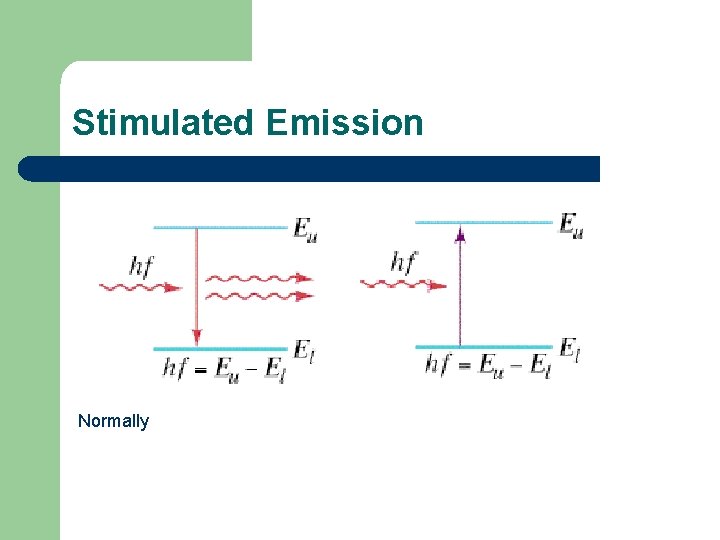
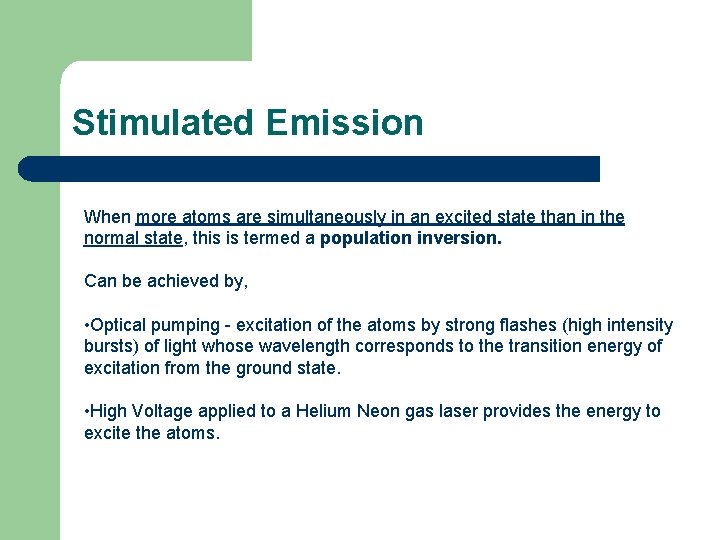
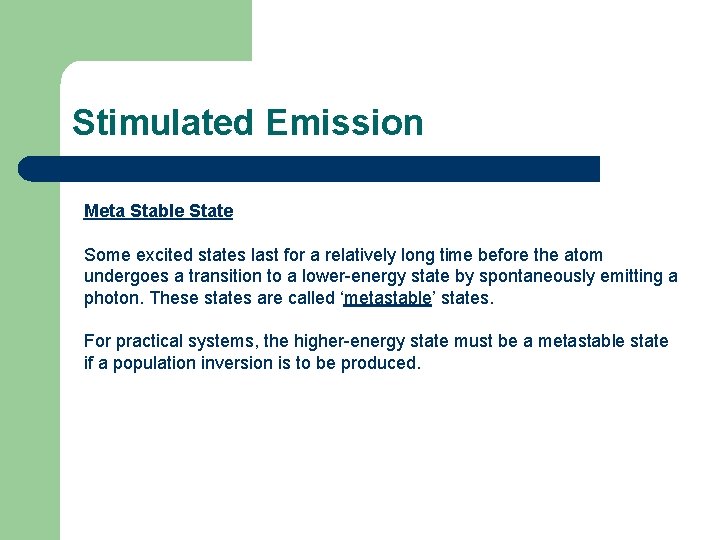
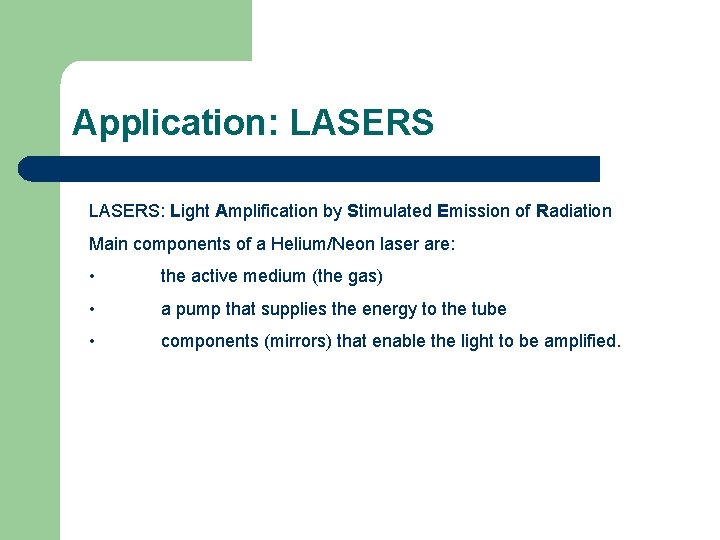
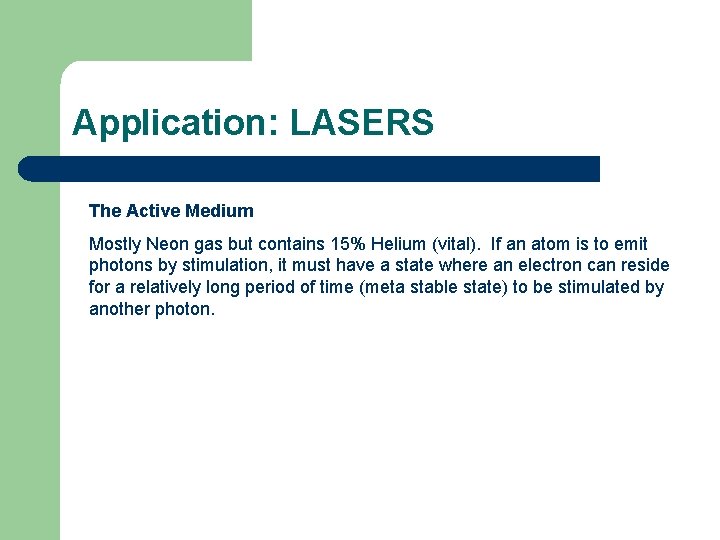
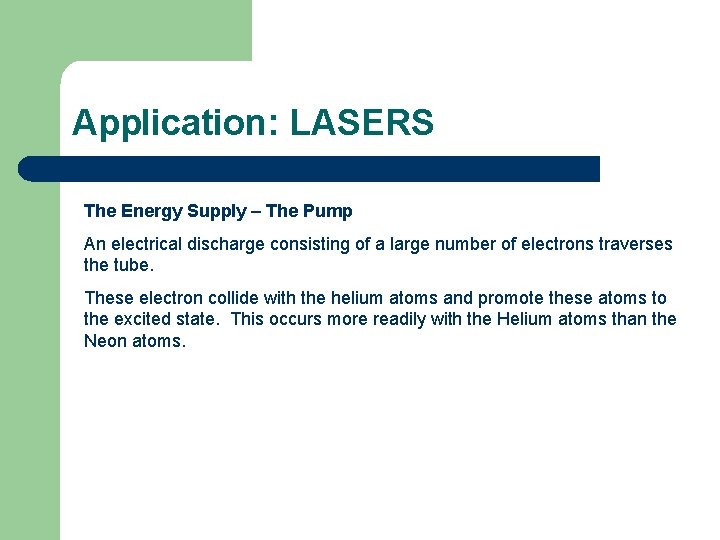
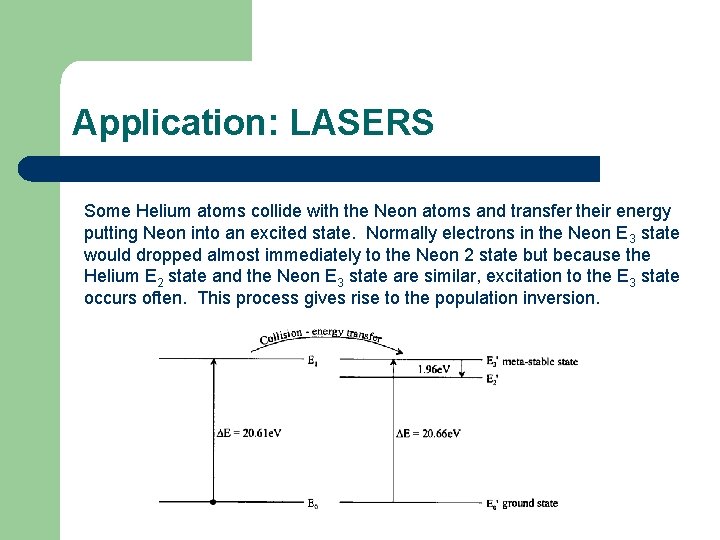
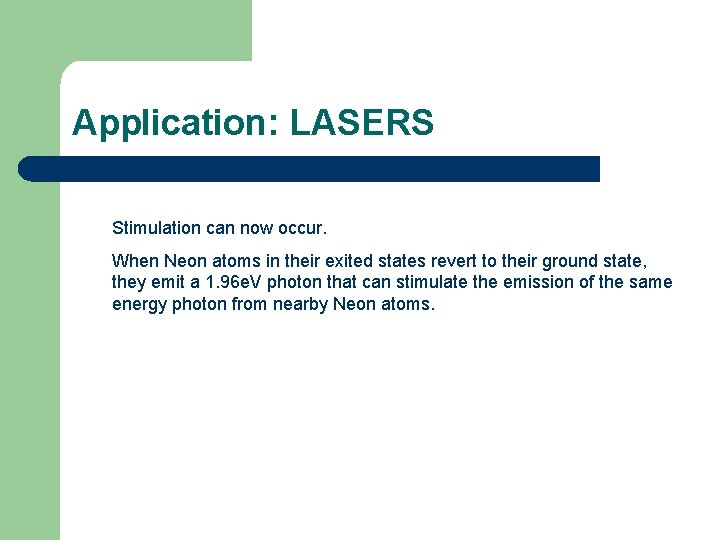
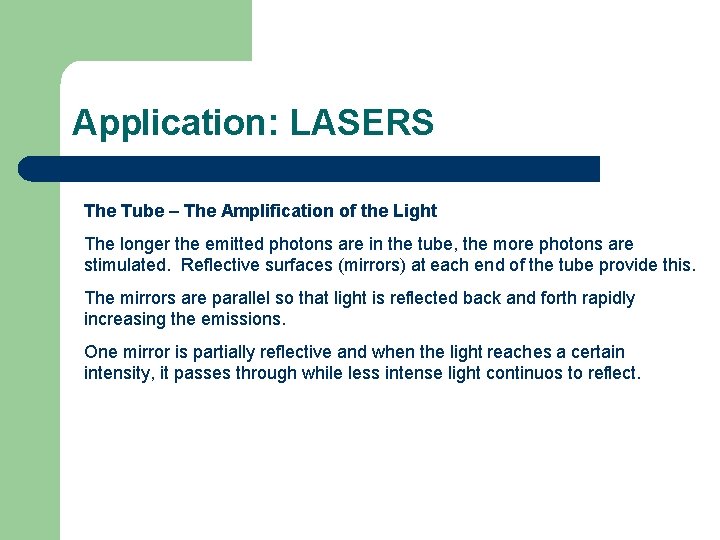
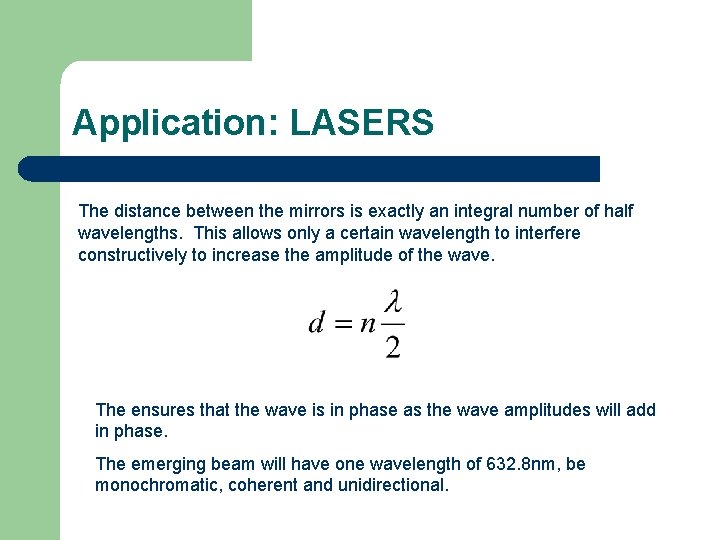
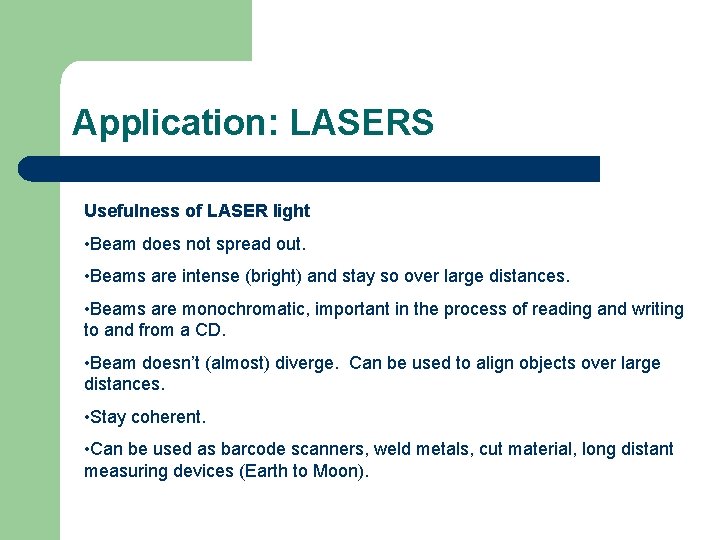
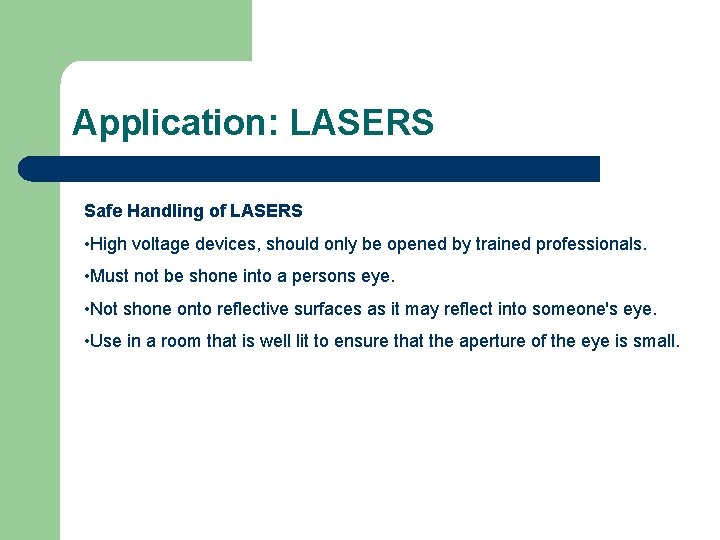
- Slides: 54
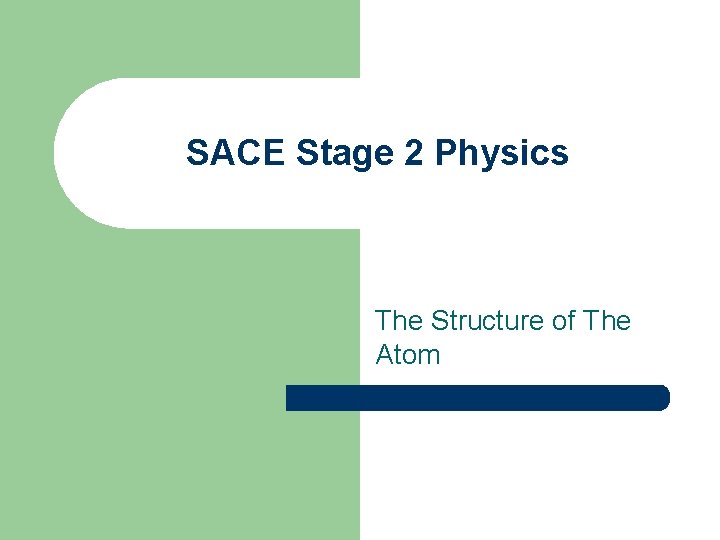
SACE Stage 2 Physics The Structure of The Atom
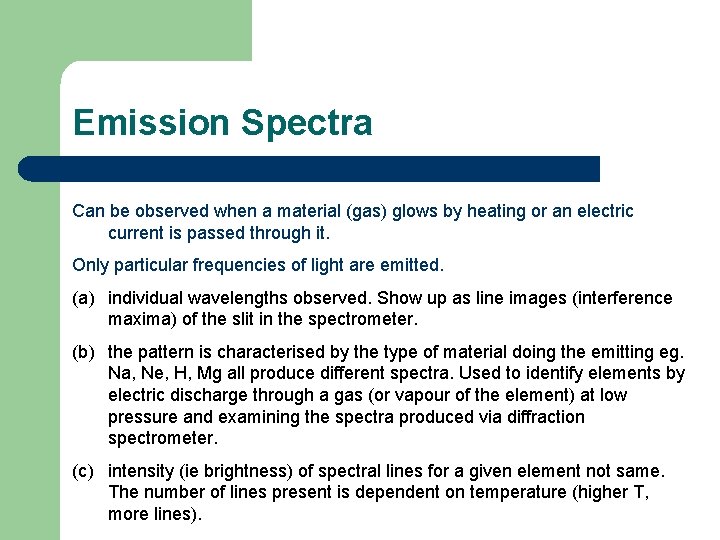
Emission Spectra Can be observed when a material (gas) glows by heating or an electric current is passed through it. Only particular frequencies of light are emitted. (a) individual wavelengths observed. Show up as line images (interference maxima) of the slit in the spectrometer. (b) the pattern is characterised by the type of material doing the emitting eg. Na, Ne, H, Mg all produce different spectra. Used to identify elements by electric discharge through a gas (or vapour of the element) at low pressure and examining the spectra produced via diffraction spectrometer. (c) intensity (ie brightness) of spectral lines for a given element not same. The number of lines present is dependent on temperature (higher T, more lines).
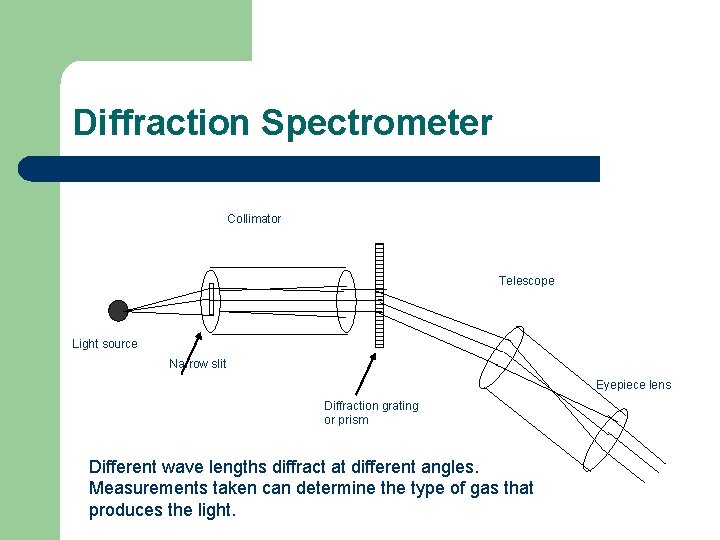
Diffraction Spectrometer Collimator Telescope Light source Narrow slit Eyepiece lens Diffraction grating or prism Different wave lengths diffract at different angles. Measurements taken can determine the type of gas that produces the light.
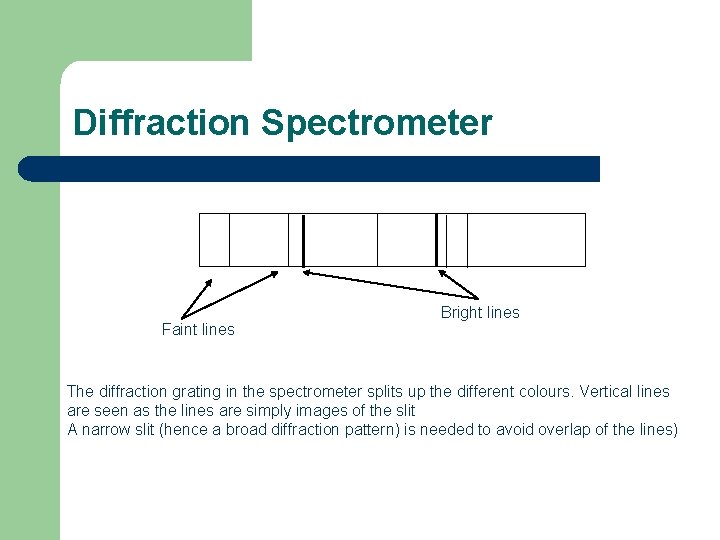
Diffraction Spectrometer Faint lines Bright lines The diffraction grating in the spectrometer splits up the different colours. Vertical lines are seen as the lines are simply images of the slit A narrow slit (hence a broad diffraction pattern) is needed to avoid overlap of the lines)
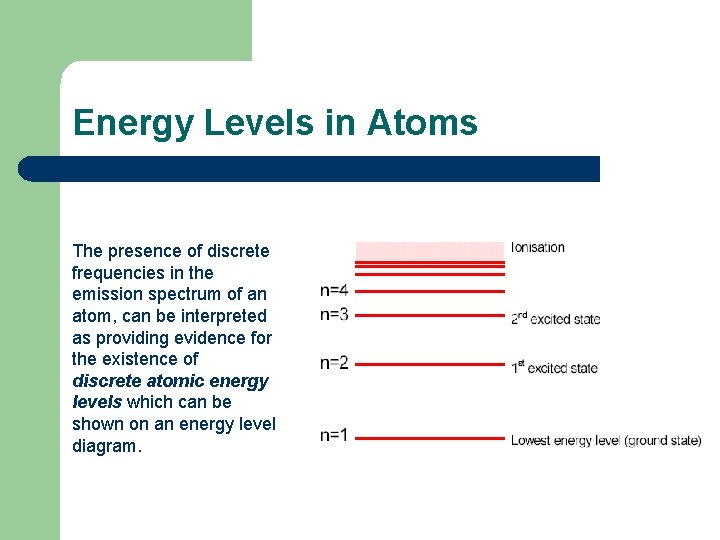
Energy Levels in Atoms The presence of discrete frequencies in the emission spectrum of an atom, can be interpreted as providing evidence for the existence of discrete atomic energy levels which can be shown on an energy level diagram.
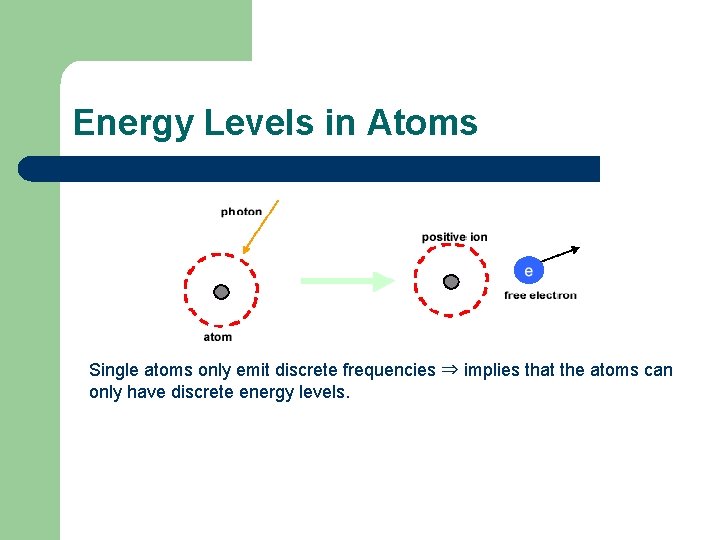
Energy Levels in Atoms Single atoms only emit discrete frequencies ⇒ implies that the atoms can only have discrete energy levels.
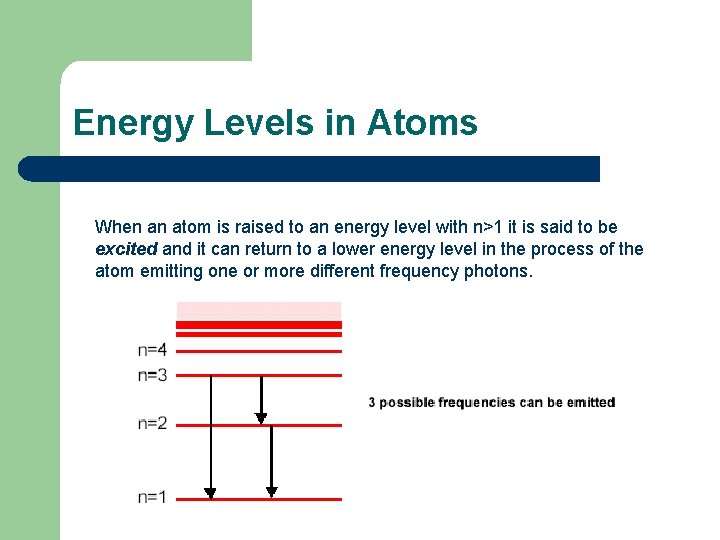
Energy Levels in Atoms When an atom is raised to an energy level with n>1 it is said to be excited and it can return to a lower energy level in the process of the atom emitting one or more different frequency photons.
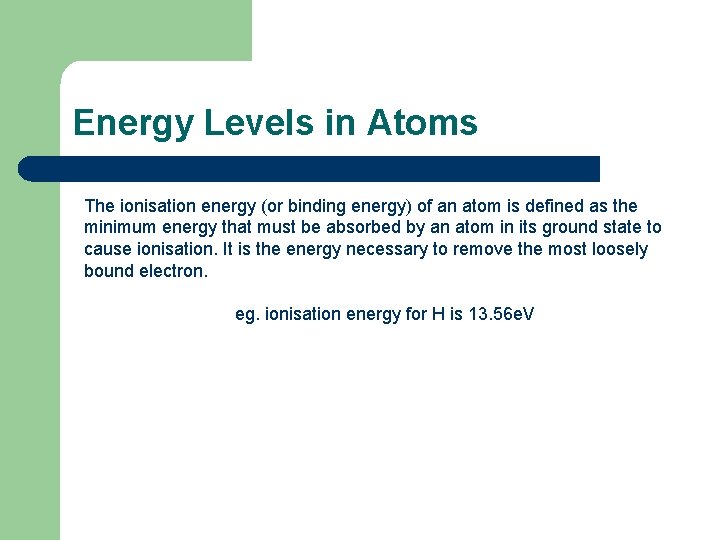
Energy Levels in Atoms The ionisation energy (or binding energy) of an atom is defined as the minimum energy that must be absorbed by an atom in its ground state to cause ionisation. It is the energy necessary to remove the most loosely bound electron. eg. ionisation energy for H is 13. 56 e. V
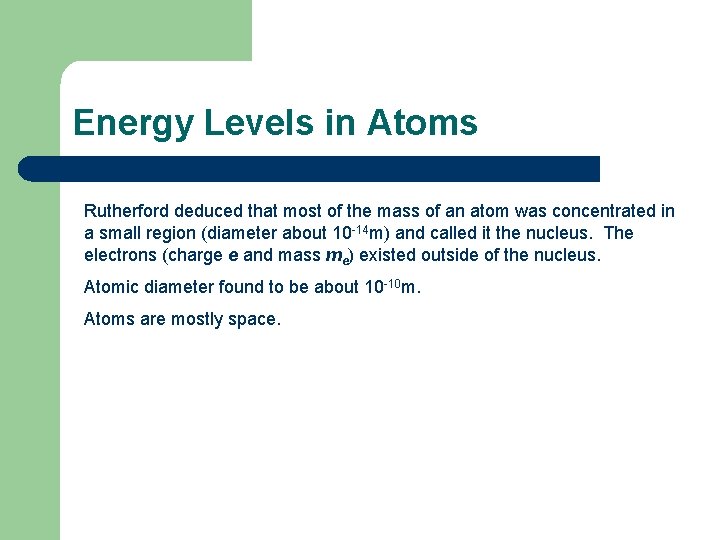
Energy Levels in Atoms Rutherford deduced that most of the mass of an atom was concentrated in a small region (diameter about 10 -14 m) and called it the nucleus. The electrons (charge e and mass me) existed outside of the nucleus. Atomic diameter found to be about 10 -10 m. Atoms are mostly space.
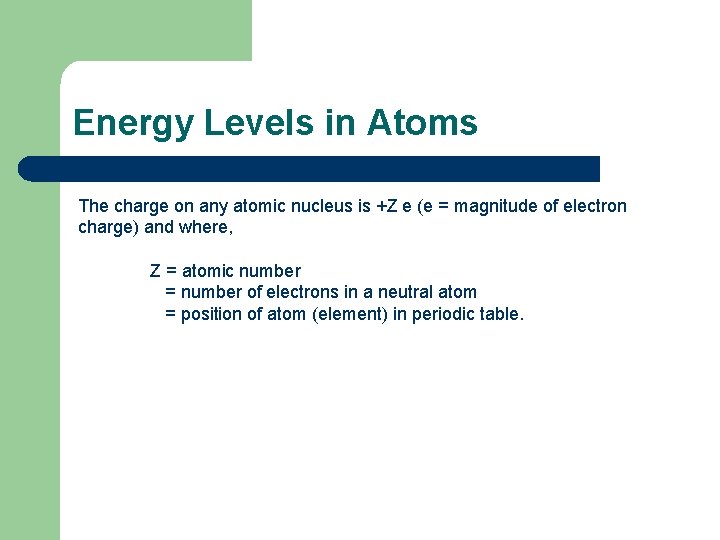
Energy Levels in Atoms The charge on any atomic nucleus is +Z e (e = magnitude of electron charge) and where, Z = atomic number = number of electrons in a neutral atom = position of atom (element) in periodic table.
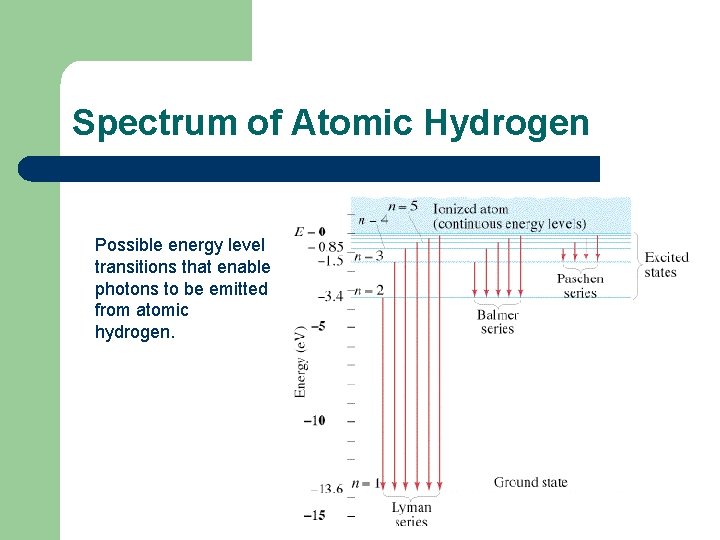
Spectrum of Atomic Hydrogen Possible energy level transitions that enable photons to be emitted from atomic hydrogen.
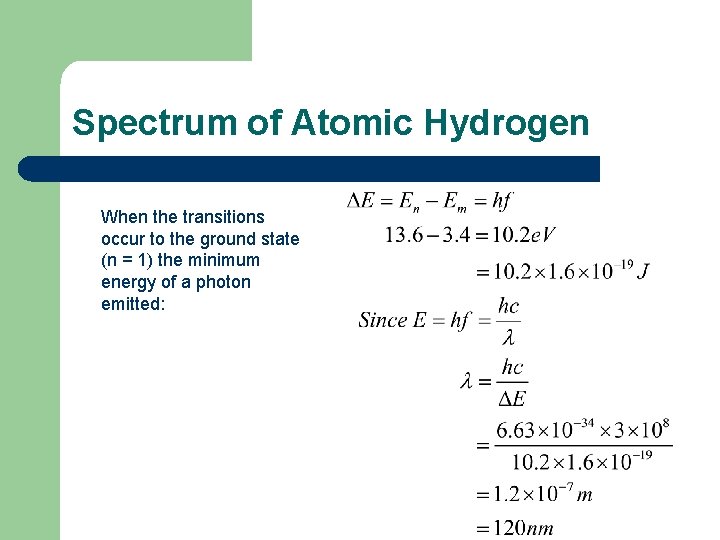
Spectrum of Atomic Hydrogen When the transitions occur to the ground state (n = 1) the minimum energy of a photon emitted:
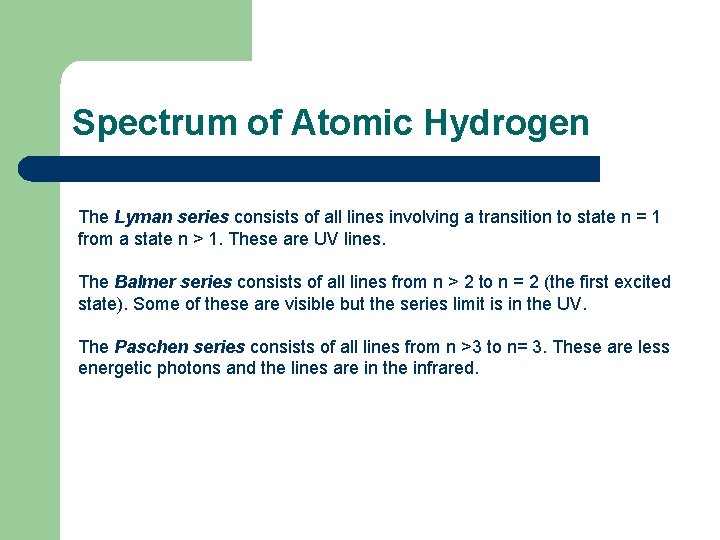
Spectrum of Atomic Hydrogen The Lyman series consists of all lines involving a transition to state n = 1 from a state n > 1. These are UV lines. The Balmer series consists of all lines from n > 2 to n = 2 (the first excited state). Some of these are visible but the series limit is in the UV. The Paschen series consists of all lines from n >3 to n= 3. These are less energetic photons and the lines are in the infrared.
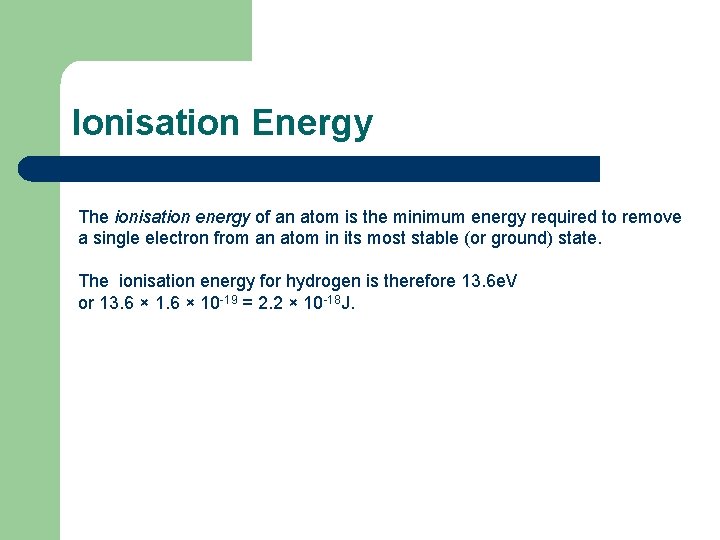
Ionisation Energy The ionisation energy of an atom is the minimum energy required to remove a single electron from an atom in its most stable (or ground) state. The ionisation energy for hydrogen is therefore 13. 6 e. V or 13. 6 × 10 -19 = 2. 2 × 10 -18 J.
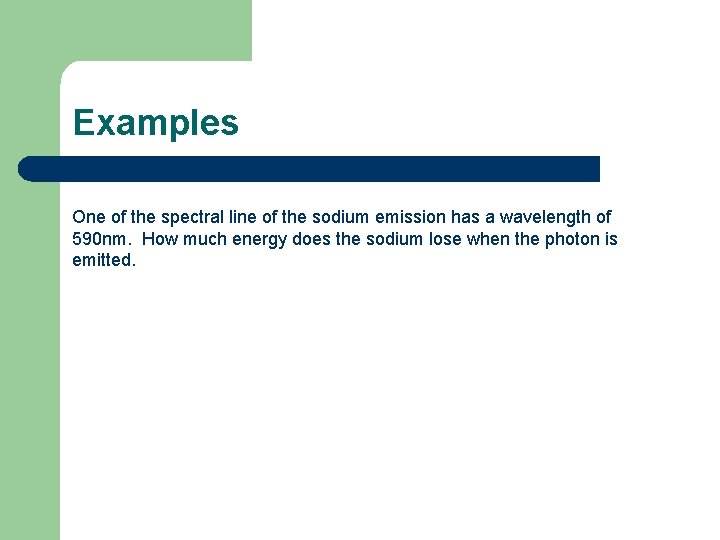
Examples One of the spectral line of the sodium emission has a wavelength of 590 nm. How much energy does the sodium lose when the photon is emitted.
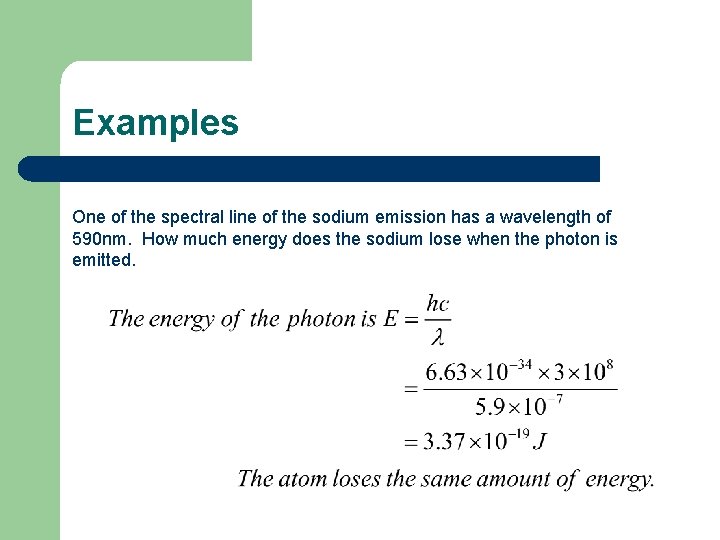
Examples One of the spectral line of the sodium emission has a wavelength of 590 nm. How much energy does the sodium lose when the photon is emitted.
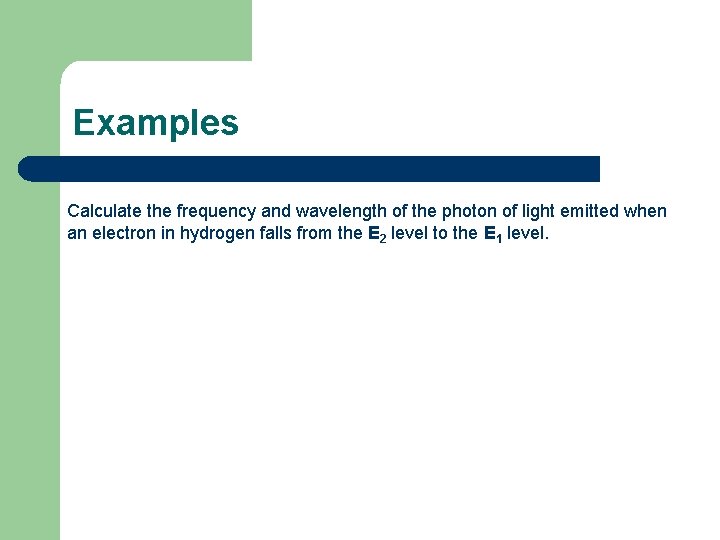
Examples Calculate the frequency and wavelength of the photon of light emitted when an electron in hydrogen falls from the E 2 level to the E 1 level.
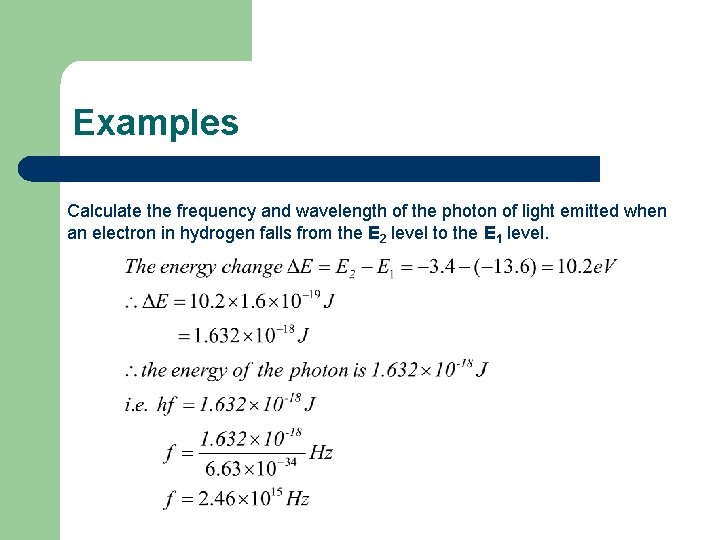
Examples Calculate the frequency and wavelength of the photon of light emitted when an electron in hydrogen falls from the E 2 level to the E 1 level.
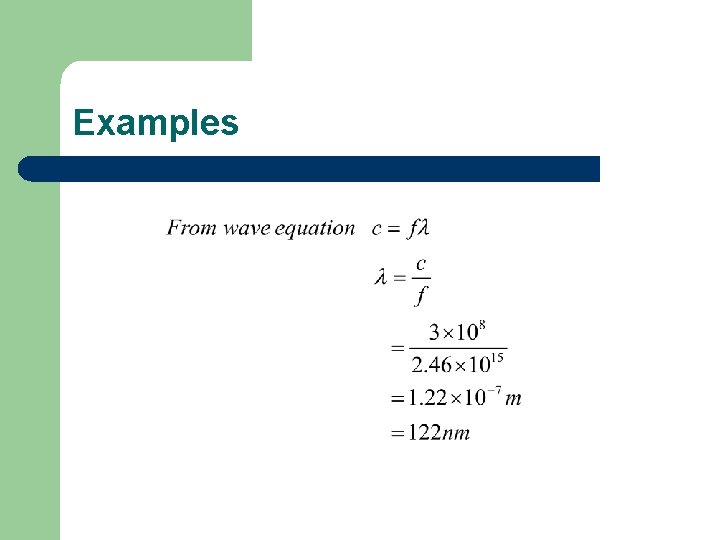
Examples
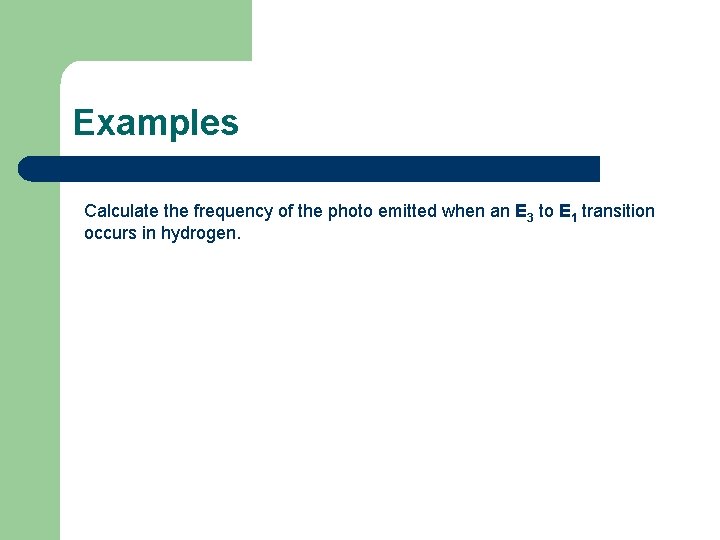
Examples Calculate the frequency of the photo emitted when an E 3 to E 1 transition occurs in hydrogen.
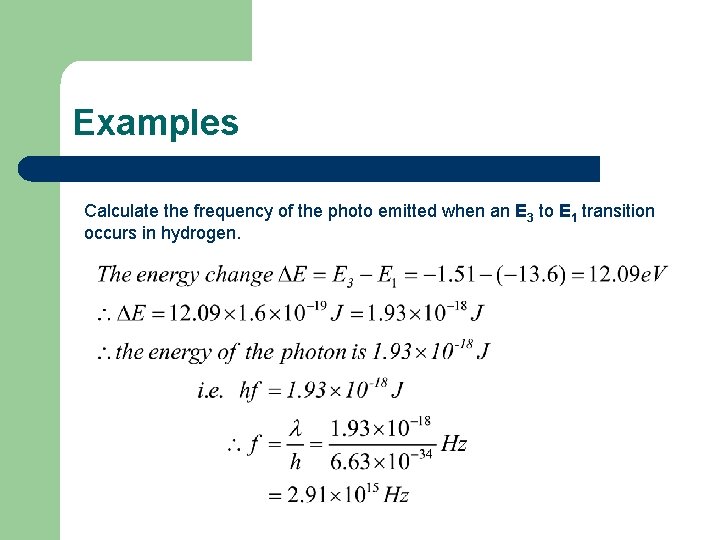
Examples Calculate the frequency of the photo emitted when an E 3 to E 1 transition occurs in hydrogen.
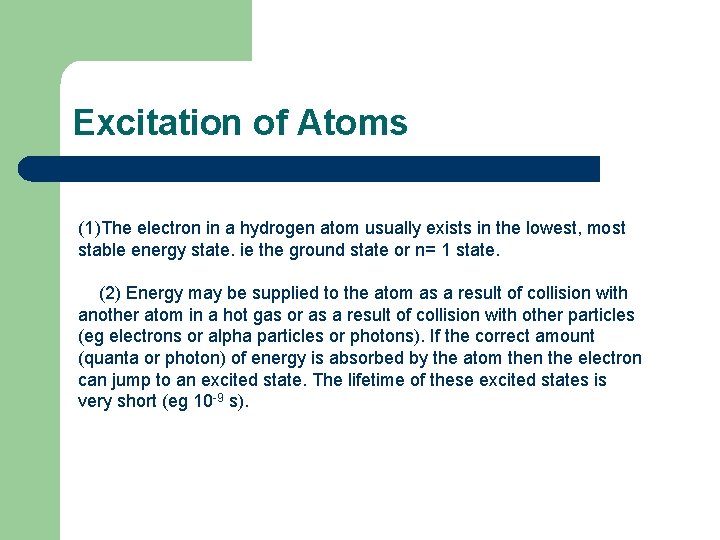
Excitation of Atoms (1)The electron in a hydrogen atom usually exists in the lowest, most stable energy state. ie the ground state or n= 1 state. (2) Energy may be supplied to the atom as a result of collision with another atom in a hot gas or as a result of collision with other particles (eg electrons or alpha particles or photons). If the correct amount (quanta or photon) of energy is absorbed by the atom then the electron can jump to an excited state. The lifetime of these excited states is very short (eg 10 -9 s).
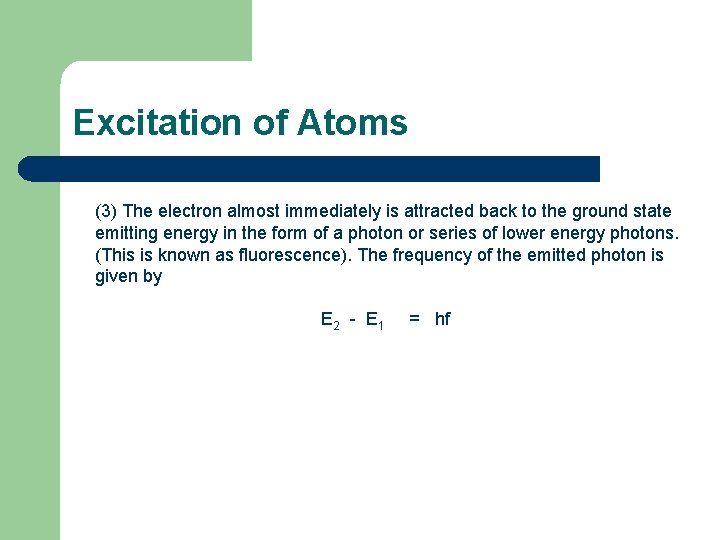
Excitation of Atoms (3) The electron almost immediately is attracted back to the ground state emitting energy in the form of a photon or series of lower energy photons. (This is known as fluorescence). The frequency of the emitted photon is given by E 2 - E 1 = hf
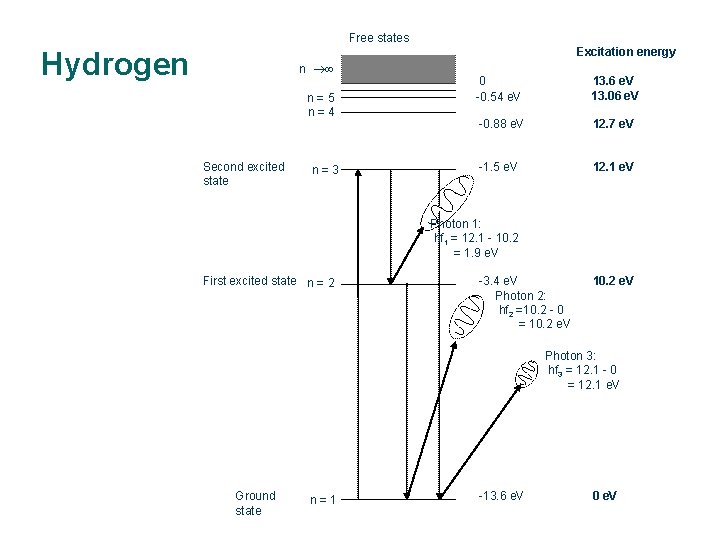
Free states Excitation energy Hydrogen n n=5 n=4 Second excited state n=3 0 -0. 54 e. V 13. 6 e. V 13. 06 e. V -0. 88 e. V 12. 7 e. V -1. 5 e. V 12. 1 e. V Photon 1: hf 1 = 12. 1 - 10. 2 = 1. 9 e. V First excited state n = 2 -3. 4 e. V Photon 2: hf 2 =10. 2 - 0 = 10. 2 e. V Photon 3: hf 3 = 12. 1 - 0 = 12. 1 e. V Ground state n=1 -13. 6 e. V 0 e. V
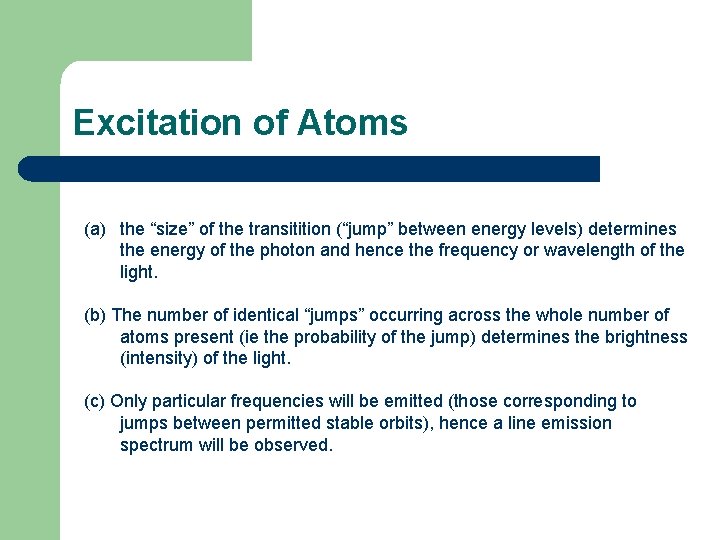
Excitation of Atoms (a) the “size” of the transitition (“jump” between energy levels) determines the energy of the photon and hence the frequency or wavelength of the light. (b) The number of identical “jumps” occurring across the whole number of atoms present (ie the probability of the jump) determines the brightness (intensity) of the light. (c) Only particular frequencies will be emitted (those corresponding to jumps between permitted stable orbits), hence a line emission spectrum will be observed.
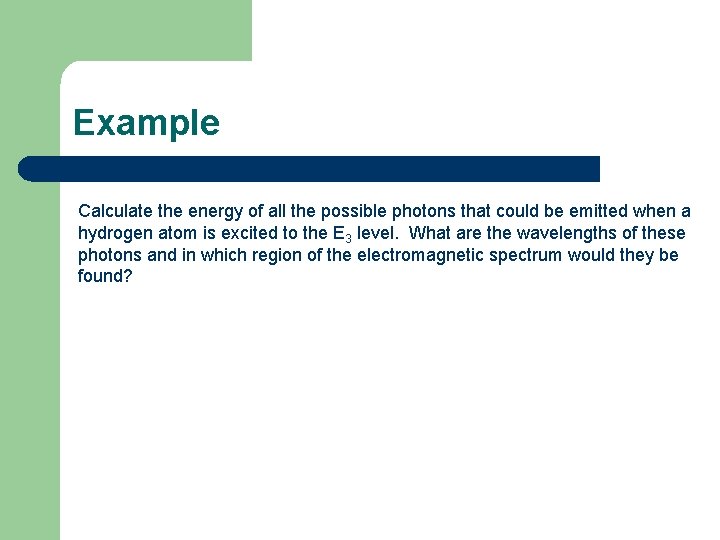
Example Calculate the energy of all the possible photons that could be emitted when a hydrogen atom is excited to the E 3 level. What are the wavelengths of these photons and in which region of the electromagnetic spectrum would they be found?
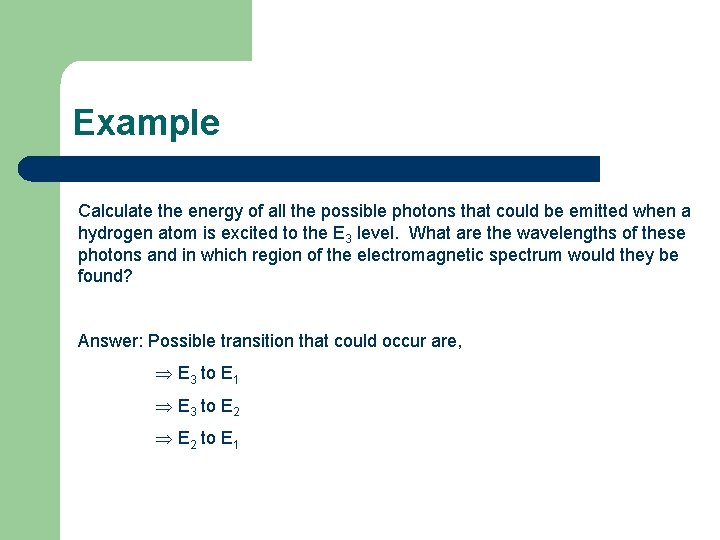
Example Calculate the energy of all the possible photons that could be emitted when a hydrogen atom is excited to the E 3 level. What are the wavelengths of these photons and in which region of the electromagnetic spectrum would they be found? Answer: Possible transition that could occur are, E 3 to E 1 E 3 to E 2 to E 1
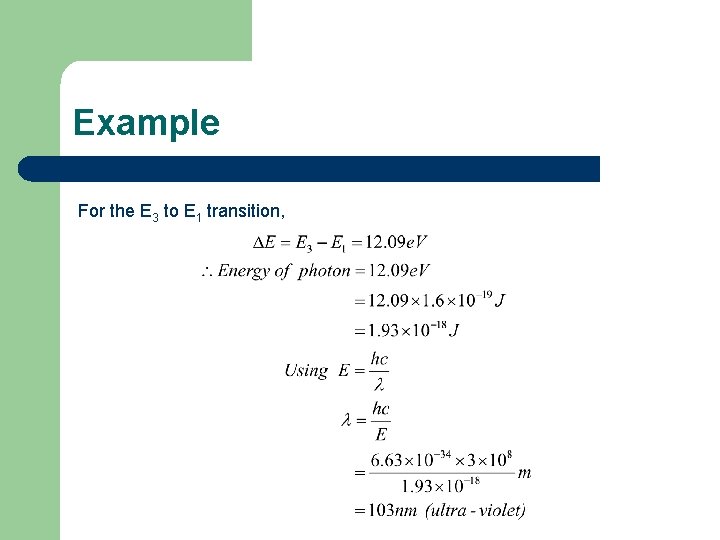
Example For the E 3 to E 1 transition,
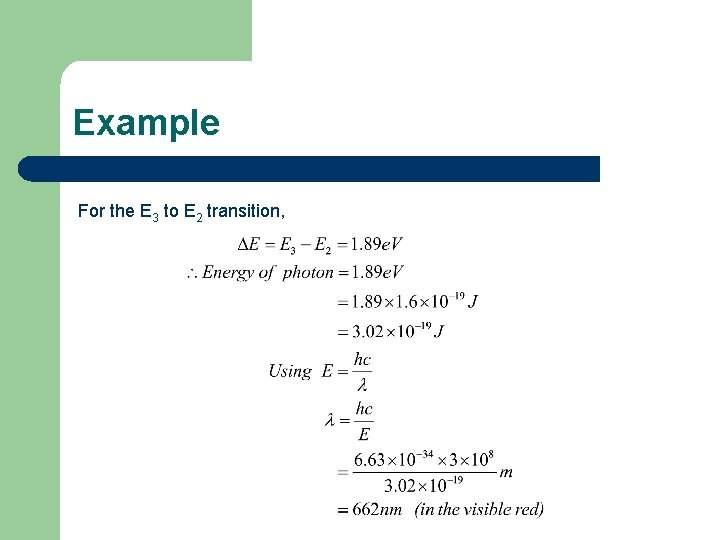
Example For the E 3 to E 2 transition,
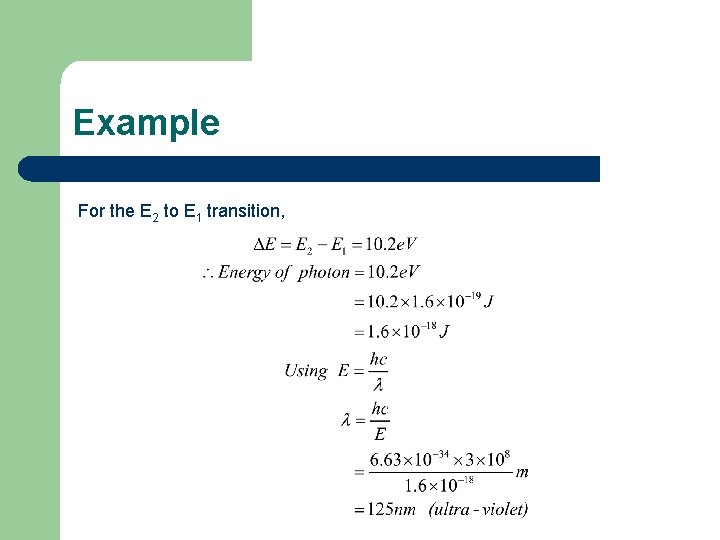
Example For the E 2 to E 1 transition,
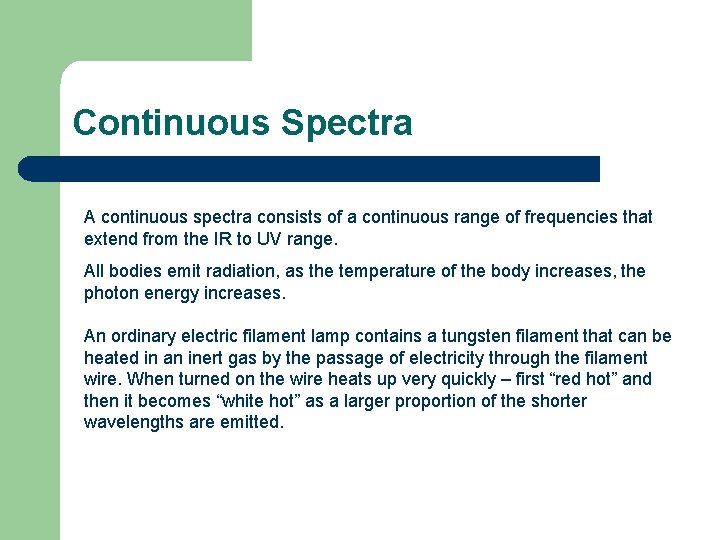
Continuous Spectra A continuous spectra consists of a continuous range of frequencies that extend from the IR to UV range. All bodies emit radiation, as the temperature of the body increases, the photon energy increases. An ordinary electric filament lamp contains a tungsten filament that can be heated in an inert gas by the passage of electricity through the filament wire. When turned on the wire heats up very quickly – first “red hot” and then it becomes “white hot” as a larger proportion of the shorter wavelengths are emitted.
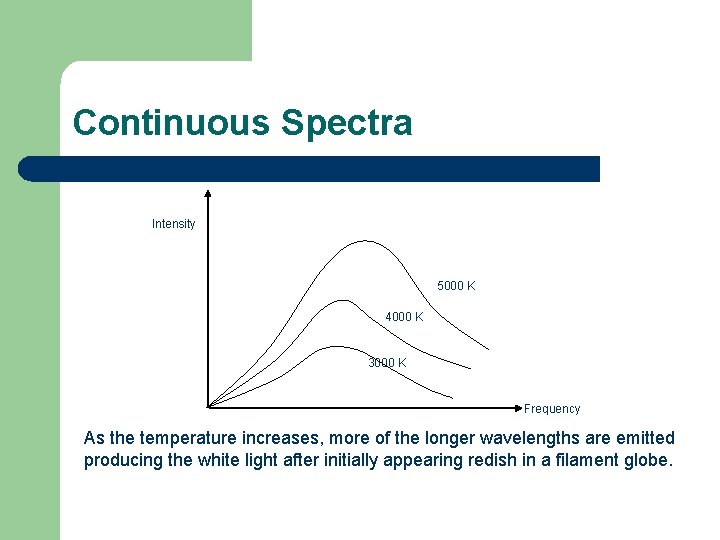
Continuous Spectra Intensity 5000 K 4000 K 3000 K Frequency As the temperature increases, more of the longer wavelengths are emitted producing the white light after initially appearing redish in a filament globe.
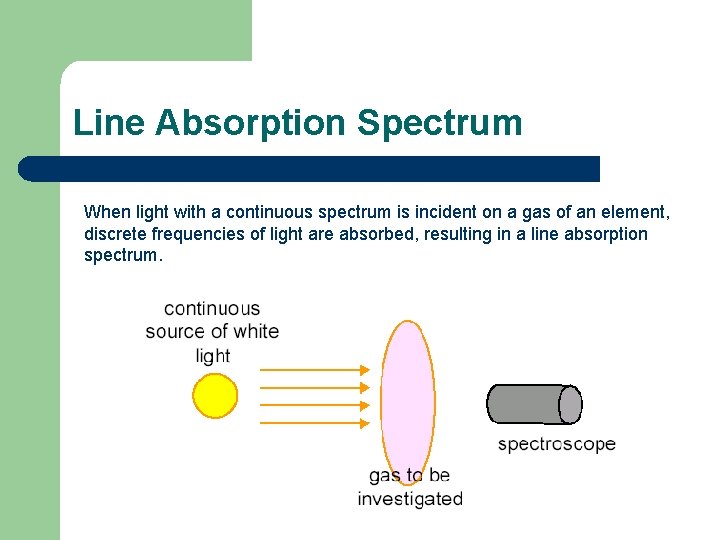
Line Absorption Spectrum When light with a continuous spectrum is incident on a gas of an element, discrete frequencies of light are absorbed, resulting in a line absorption spectrum.
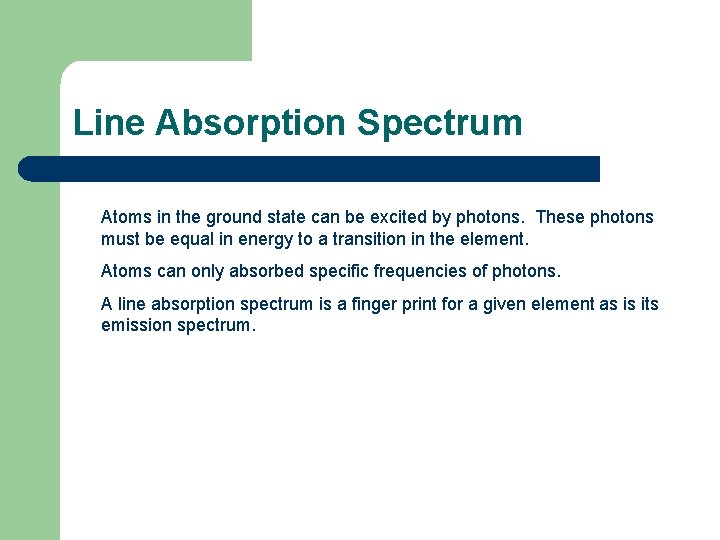
Line Absorption Spectrum Atoms in the ground state can be excited by photons. These photons must be equal in energy to a transition in the element. Atoms can only absorbed specific frequencies of photons. A line absorption spectrum is a finger print for a given element as is its emission spectrum.
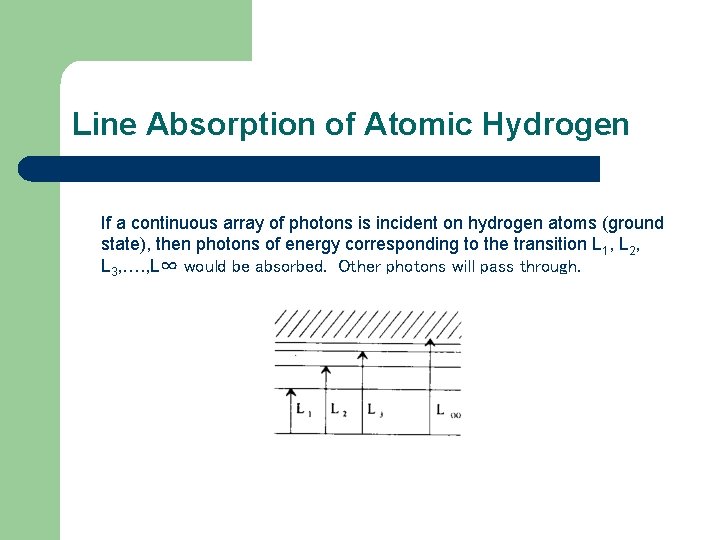
Line Absorption of Atomic Hydrogen If a continuous array of photons is incident on hydrogen atoms (ground state), then photons of energy corresponding to the transition L 1, L 2, L 3, …. , L∞ would be absorbed. Other photons will pass through.
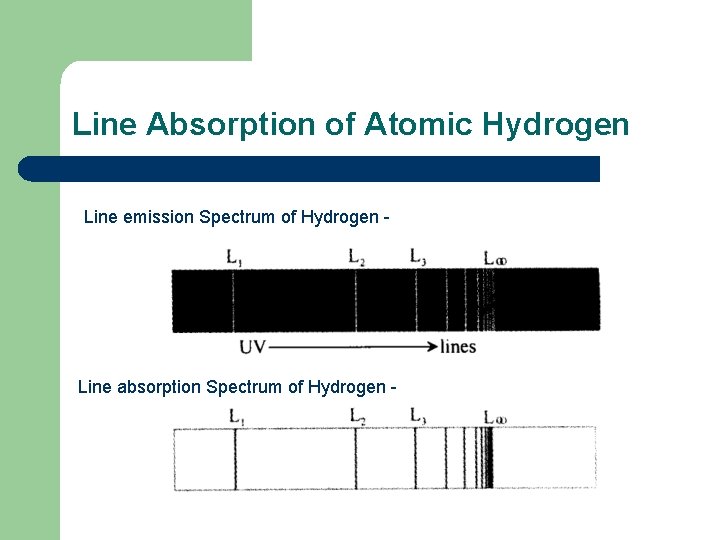
Line Absorption of Atomic Hydrogen Line emission Spectrum of Hydrogen - Line absorption Spectrum of Hydrogen -
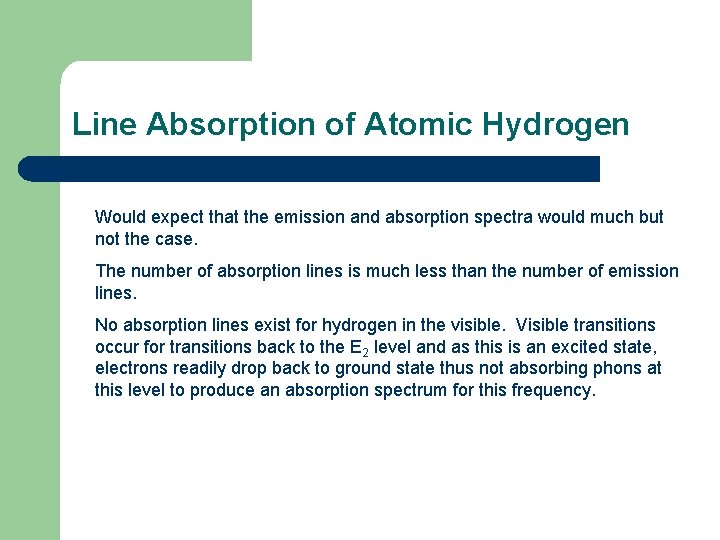
Line Absorption of Atomic Hydrogen Would expect that the emission and absorption spectra would much but not the case. The number of absorption lines is much less than the number of emission lines. No absorption lines exist for hydrogen in the visible. Visible transitions occur for transitions back to the E 2 level and as this is an excited state, electrons readily drop back to ground state thus not absorbing phons at this level to produce an absorption spectrum for this frequency.
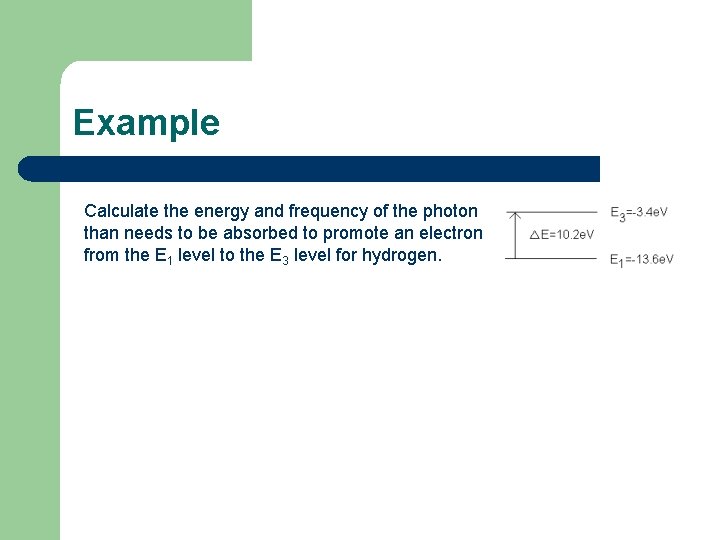
Example Calculate the energy and frequency of the photon than needs to be absorbed to promote an electron from the E 1 level to the E 3 level for hydrogen.
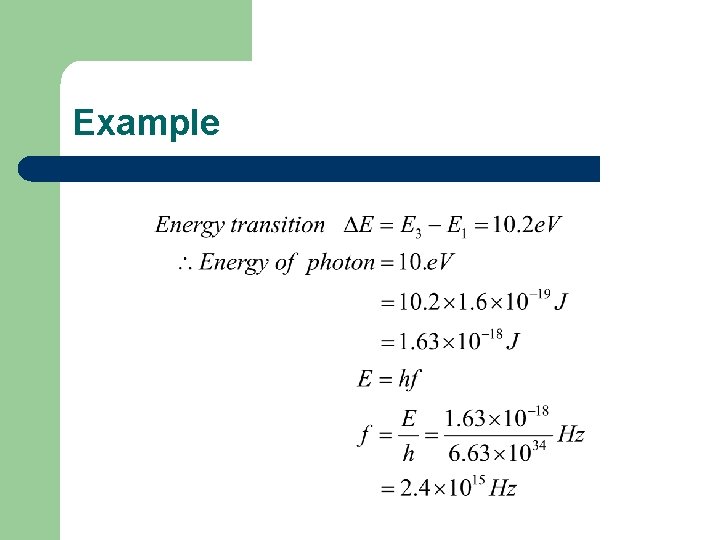
Example
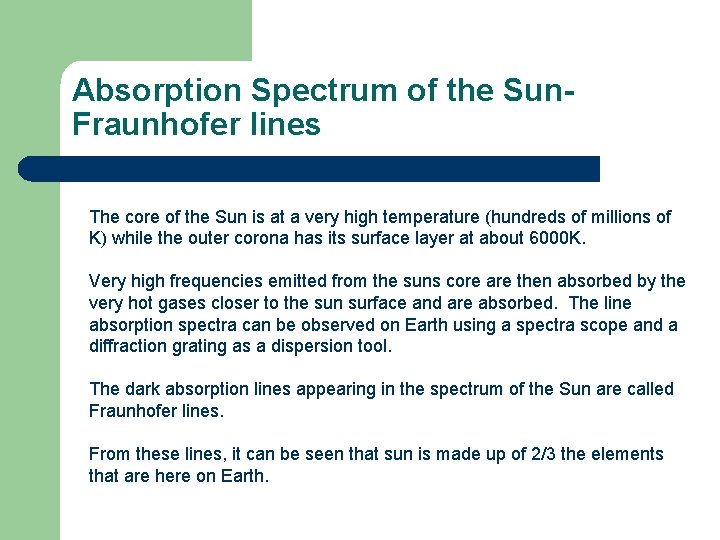
Absorption Spectrum of the Sun. Fraunhofer lines The core of the Sun is at a very high temperature (hundreds of millions of K) while the outer corona has its surface layer at about 6000 K. Very high frequencies emitted from the suns core are then absorbed by the very hot gases closer to the sun surface and are absorbed. The line absorption spectra can be observed on Earth using a spectra scope and a diffraction grating as a dispersion tool. The dark absorption lines appearing in the spectrum of the Sun are called Fraunhofer lines. From these lines, it can be seen that sun is made up of 2/3 the elements that are here on Earth.
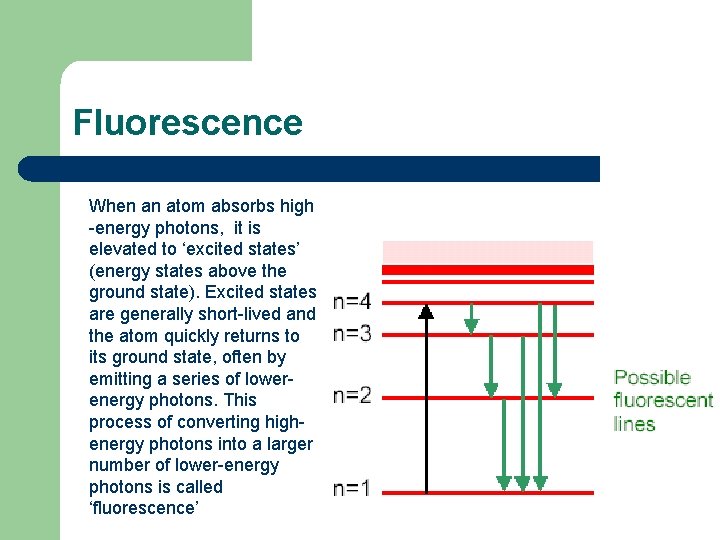
Fluorescence When an atom absorbs high -energy photons, it is elevated to ‘excited states’ (energy states above the ground state). Excited states are generally short-lived and the atom quickly returns to its ground state, often by emitting a series of lowerenergy photons. This process of converting highenergy photons into a larger number of lower-energy photons is called ‘fluorescence’
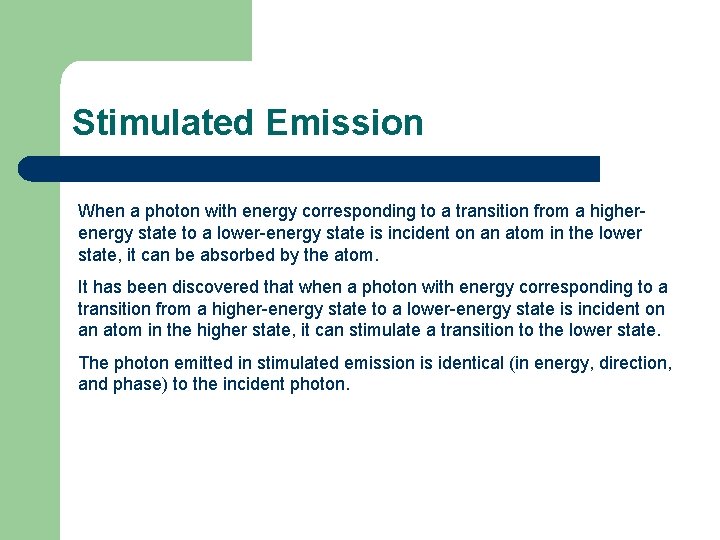
Stimulated Emission When a photon with energy corresponding to a transition from a higherenergy state to a lower-energy state is incident on an atom in the lower state, it can be absorbed by the atom. It has been discovered that when a photon with energy corresponding to a transition from a higher-energy state to a lower-energy state is incident on an atom in the higher state, it can stimulate a transition to the lower state. The photon emitted in stimulated emission is identical (in energy, direction, and phase) to the incident photon.
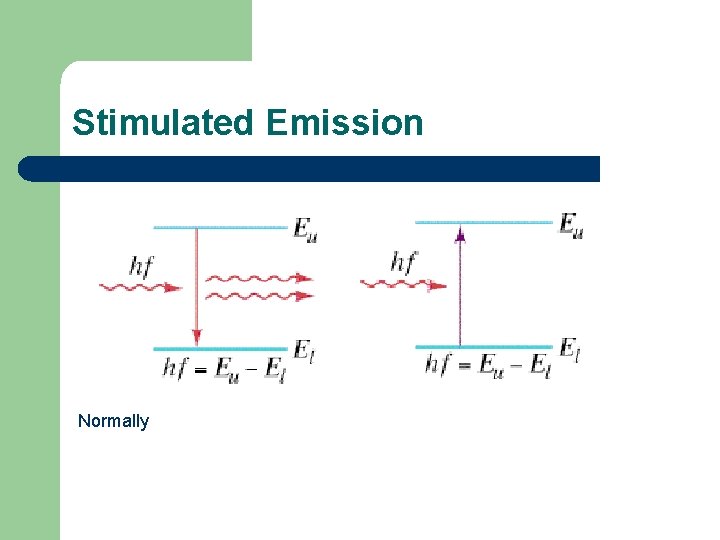
Stimulated Emission Normally
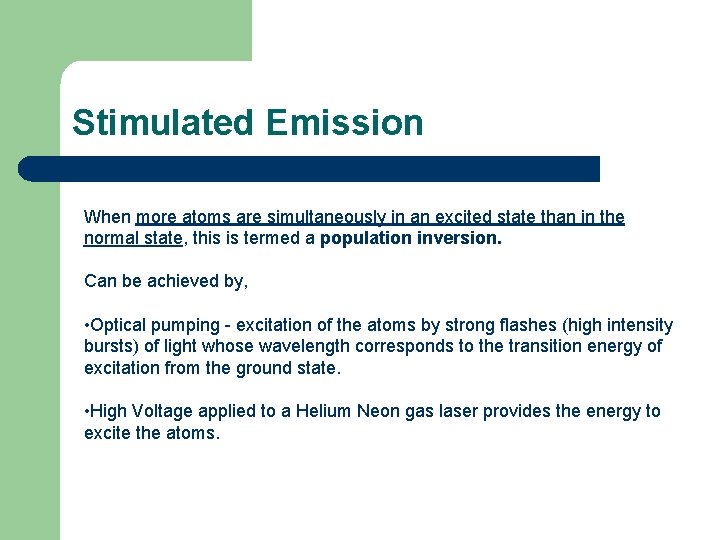
Stimulated Emission When more atoms are simultaneously in an excited state than in the normal state, this is termed a population inversion. Can be achieved by, • Optical pumping - excitation of the atoms by strong flashes (high intensity bursts) of light whose wavelength corresponds to the transition energy of excitation from the ground state. • High Voltage applied to a Helium Neon gas laser provides the energy to excite the atoms.
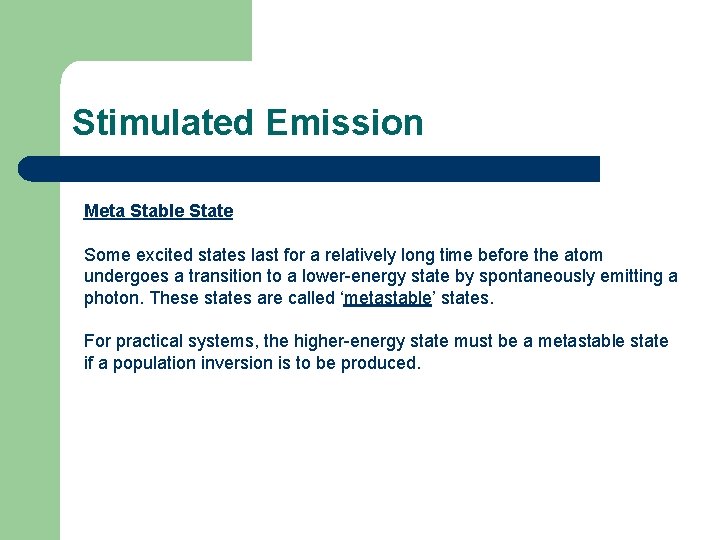
Stimulated Emission Meta Stable State Some excited states last for a relatively long time before the atom undergoes a transition to a lower-energy state by spontaneously emitting a photon. These states are called ‘metastable’ states. For practical systems, the higher-energy state must be a metastable state if a population inversion is to be produced.
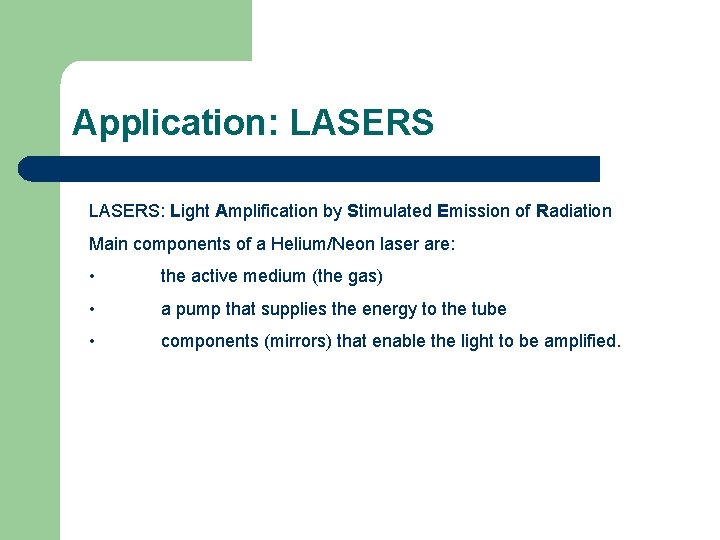
Application: LASERS: Light Amplification by Stimulated Emission of Radiation Main components of a Helium/Neon laser are: • the active medium (the gas) • a pump that supplies the energy to the tube • components (mirrors) that enable the light to be amplified.
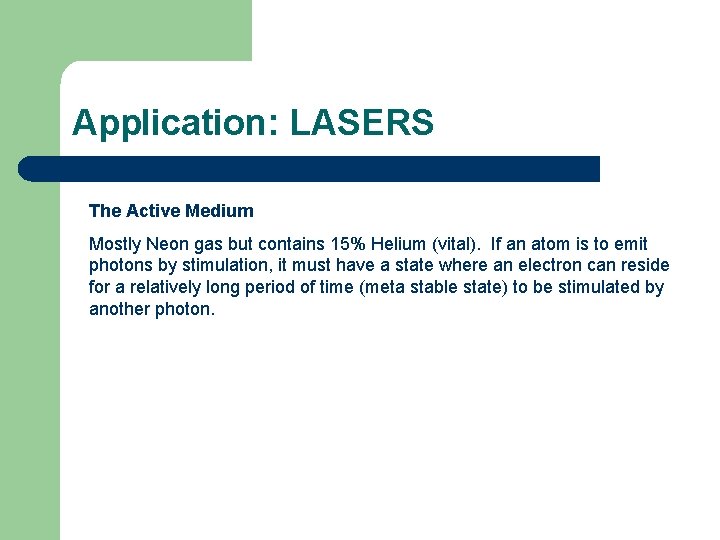
Application: LASERS The Active Medium Mostly Neon gas but contains 15% Helium (vital). If an atom is to emit photons by stimulation, it must have a state where an electron can reside for a relatively long period of time (meta stable state) to be stimulated by another photon.
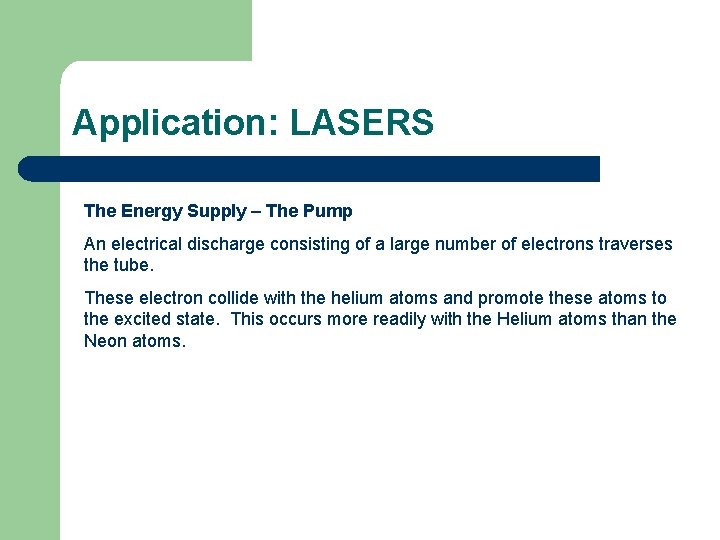
Application: LASERS The Energy Supply – The Pump An electrical discharge consisting of a large number of electrons traverses the tube. These electron collide with the helium atoms and promote these atoms to the excited state. This occurs more readily with the Helium atoms than the Neon atoms.
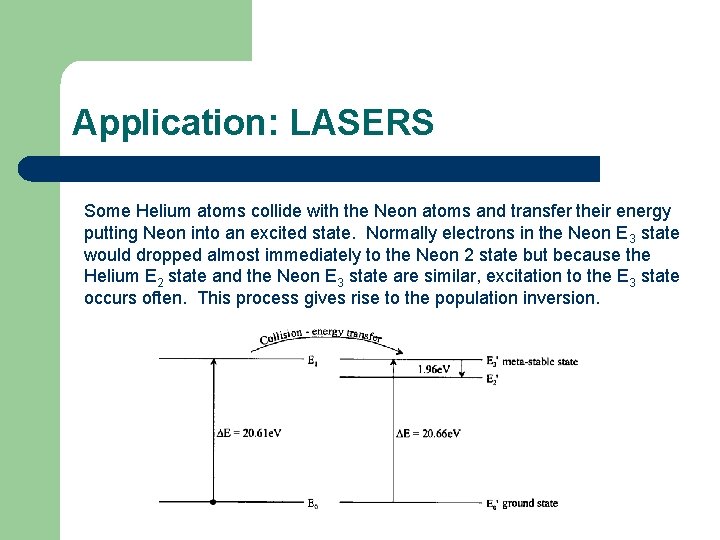
Application: LASERS Some Helium atoms collide with the Neon atoms and transfer their energy putting Neon into an excited state. Normally electrons in the Neon E 3 state would dropped almost immediately to the Neon 2 state but because the Helium E 2 state and the Neon E 3 state are similar, excitation to the E 3 state occurs often. This process gives rise to the population inversion.
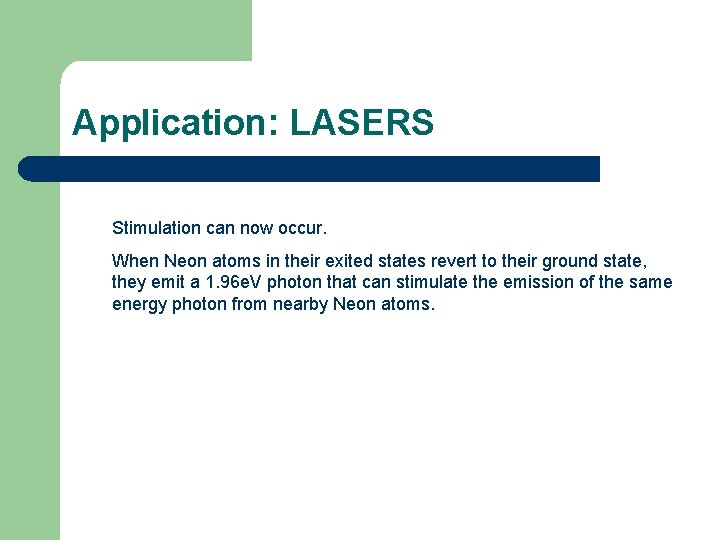
Application: LASERS Stimulation can now occur. When Neon atoms in their exited states revert to their ground state, they emit a 1. 96 e. V photon that can stimulate the emission of the same energy photon from nearby Neon atoms.
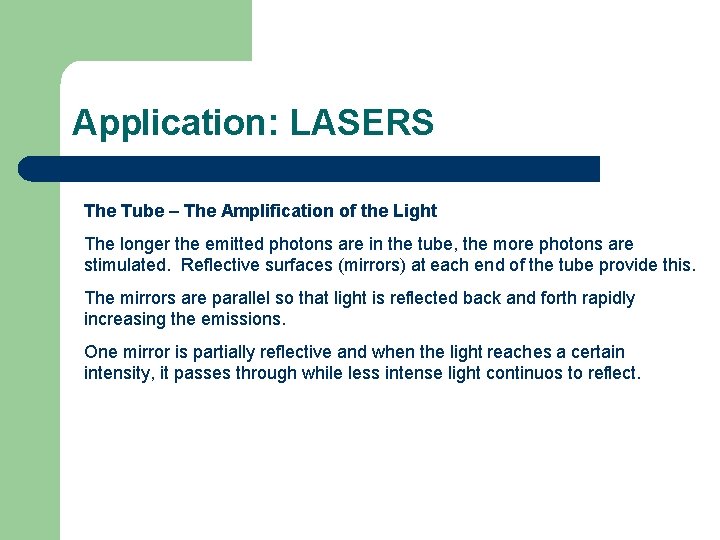
Application: LASERS The Tube – The Amplification of the Light The longer the emitted photons are in the tube, the more photons are stimulated. Reflective surfaces (mirrors) at each end of the tube provide this. The mirrors are parallel so that light is reflected back and forth rapidly increasing the emissions. One mirror is partially reflective and when the light reaches a certain intensity, it passes through while less intense light continuos to reflect.
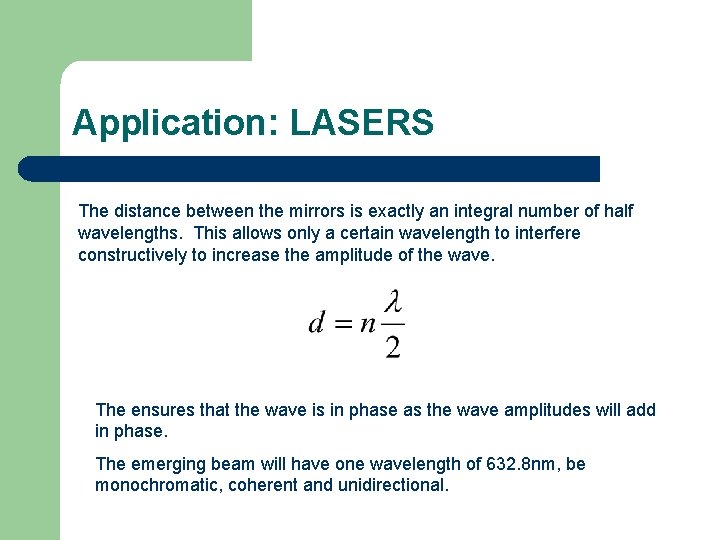
Application: LASERS The distance between the mirrors is exactly an integral number of half wavelengths. This allows only a certain wavelength to interfere constructively to increase the amplitude of the wave. The ensures that the wave is in phase as the wave amplitudes will add in phase. The emerging beam will have one wavelength of 632. 8 nm, be monochromatic, coherent and unidirectional.
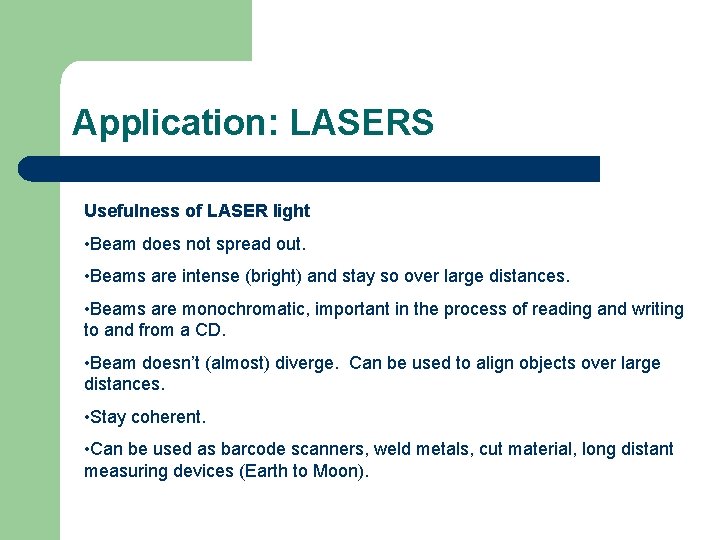
Application: LASERS Usefulness of LASER light • Beam does not spread out. • Beams are intense (bright) and stay so over large distances. • Beams are monochromatic, important in the process of reading and writing to and from a CD. • Beam doesn’t (almost) diverge. Can be used to align objects over large distances. • Stay coherent. • Can be used as barcode scanners, weld metals, cut material, long distant measuring devices (Earth to Moon).
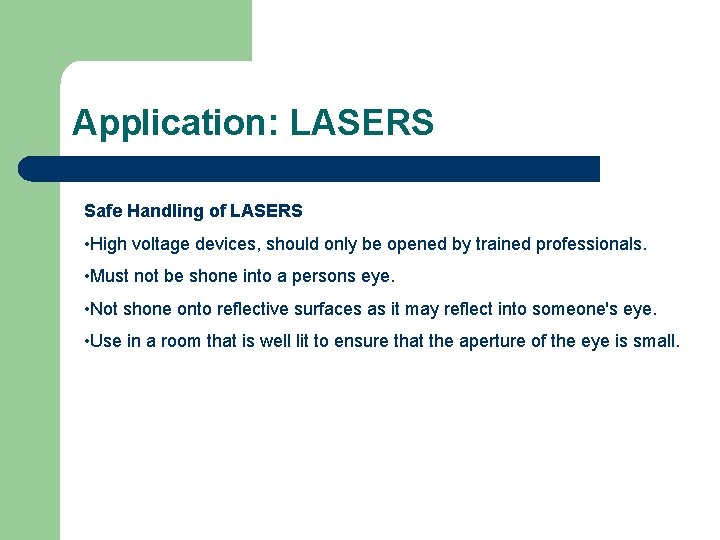
Application: LASERS Safe Handling of LASERS • High voltage devices, should only be opened by trained professionals. • Must not be shone into a persons eye. • Not shone onto reflective surfaces as it may reflect into someone's eye. • Use in a room that is well lit to ensure that the aperture of the eye is small.
Davisson germer experiment
Sace stage 2 physics
Sace stage 1 physics
Sace stage 2
Sace aggregate
Cptd
Plato sace
Screen 3
Scheda paese sace
Sace english literary studies
Sace annual report
Sace center
Sace export plus
Stage 2 denial
Staging theatre definition
A stage where the audience sits on one side only
What is a two stage tender
Downstage and upstage
Stage right and left
Modern physics vs classical physics
University physics with modern physics fifteenth edition
Example ib physics ia
La structure d'un rapport
Structure of plays
Hình ảnh bộ gõ cơ thể búng tay
Lp html
Bổ thể
Tỉ lệ cơ thể trẻ em
Gấu đi như thế nào
Tư thế worm breton là gì
Hát lên người ơi alleluia
Môn thể thao bắt đầu bằng từ chạy
Thế nào là hệ số cao nhất
Các châu lục và đại dương trên thế giới
Cong thức tính động năng
Trời xanh đây là của chúng ta thể thơ
Mật thư tọa độ 5x5
101012 bằng
độ dài liên kết
Các châu lục và đại dương trên thế giới
Thơ thất ngôn tứ tuyệt đường luật
Quá trình desamine hóa có thể tạo ra
Một số thể thơ truyền thống
Cái miệng bé xinh thế chỉ nói điều hay thôi
Vẽ hình chiếu vuông góc của vật thể sau
Thế nào là sự mỏi cơ
đặc điểm cơ thể của người tối cổ
Thế nào là giọng cùng tên? *
Vẽ hình chiếu đứng bằng cạnh của vật thể
Phối cảnh
Thẻ vin
đại từ thay thế
điện thế nghỉ
Tư thế ngồi viết
Diễn thế sinh thái là