Chapter 16 Spontaneity Entropy and Free Energy DE
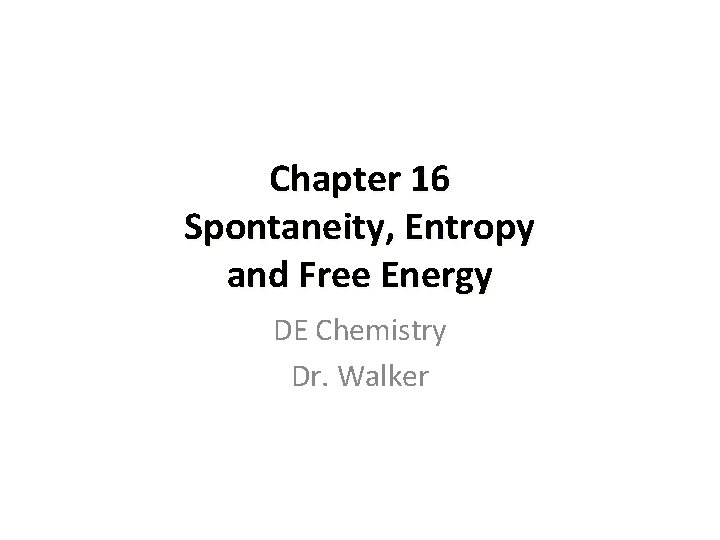
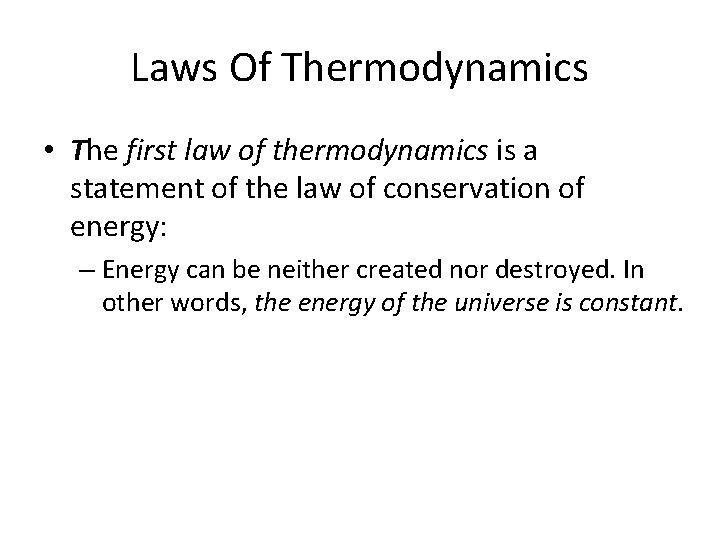
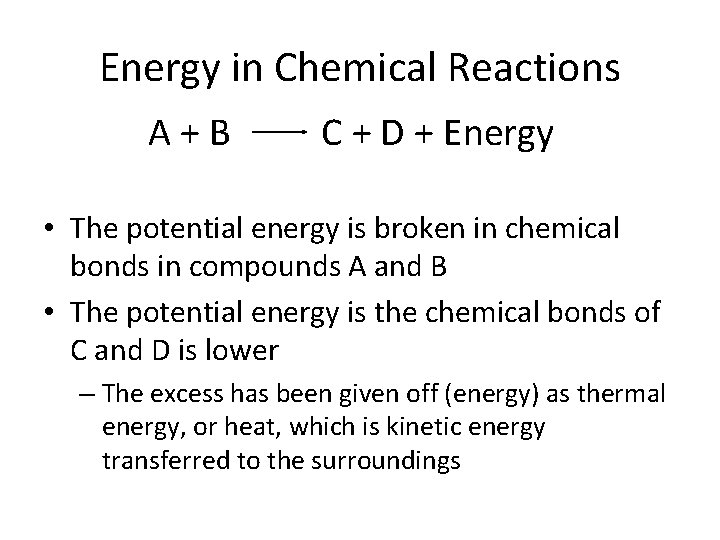
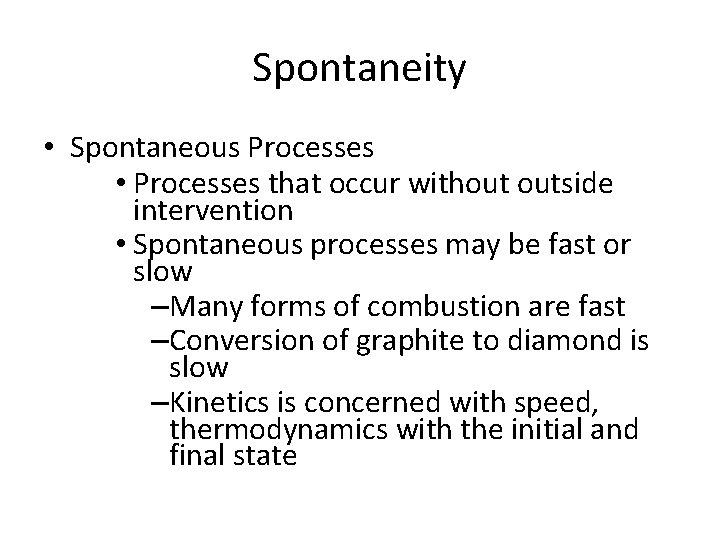
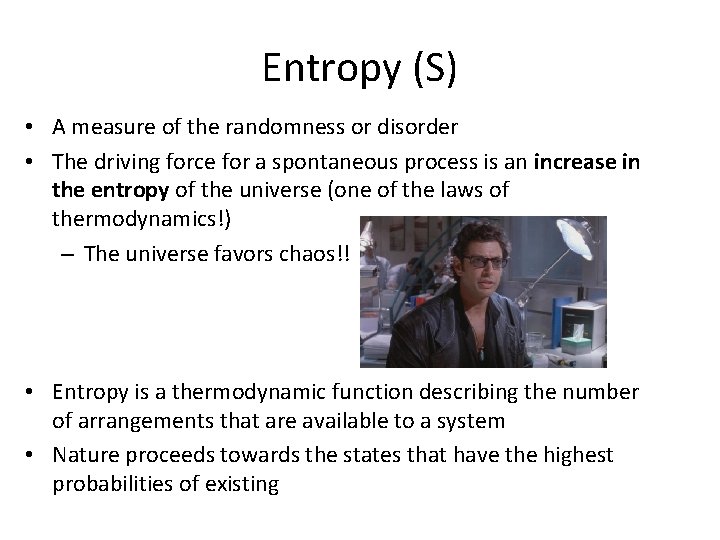
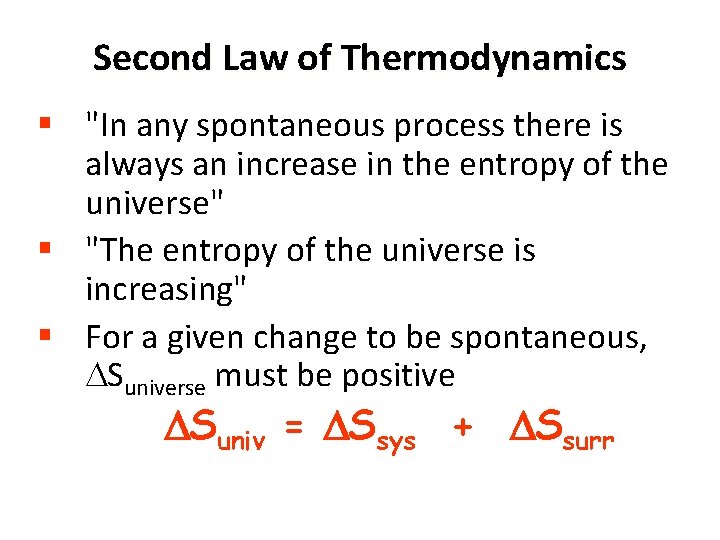
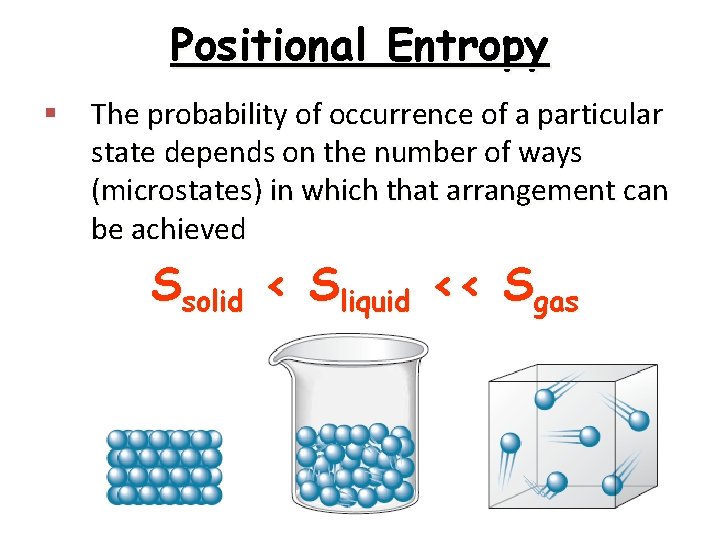
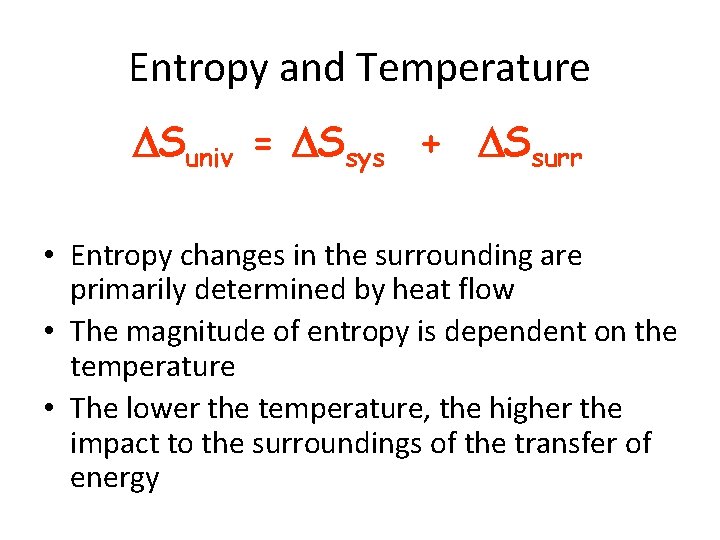
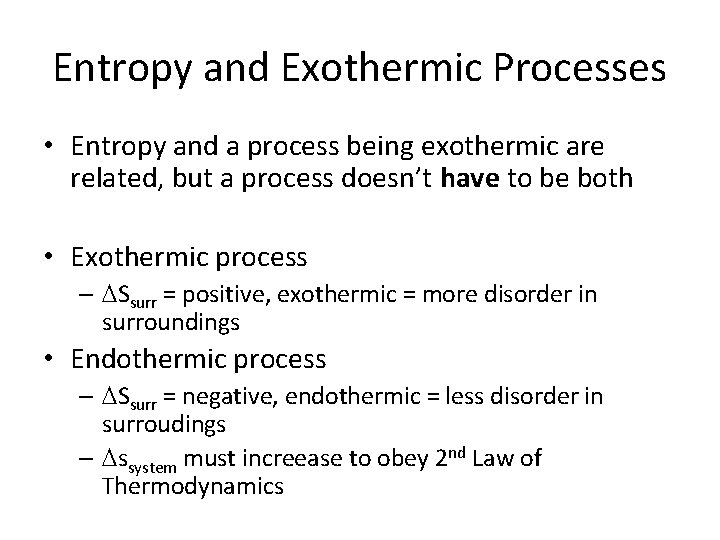
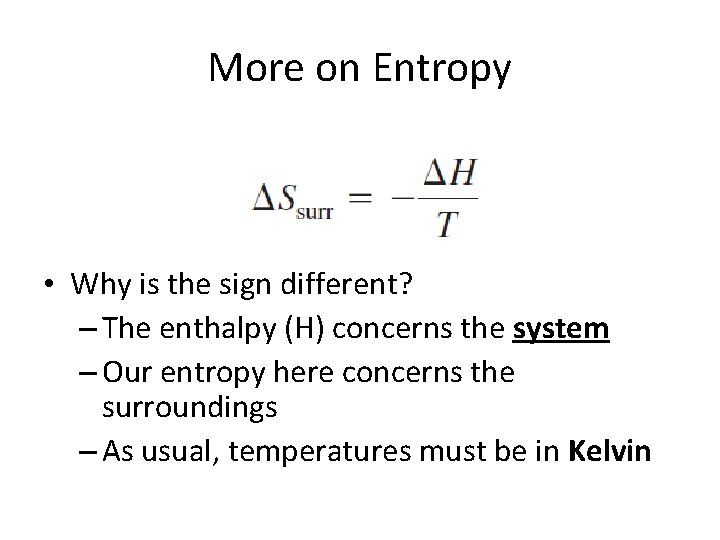
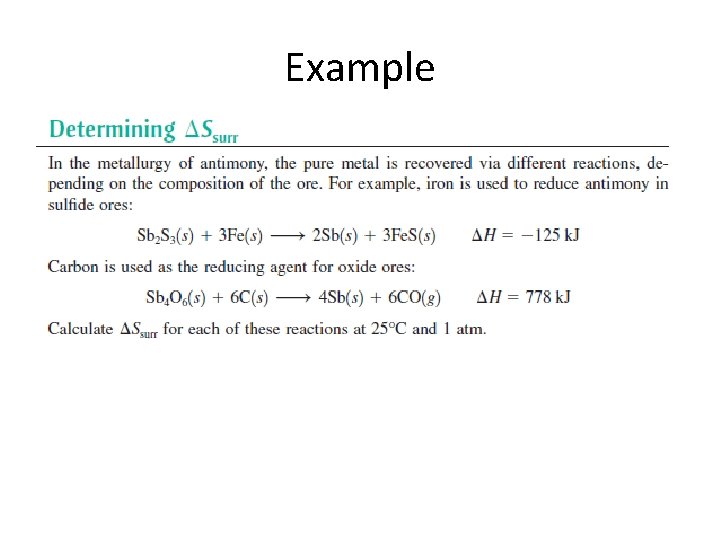
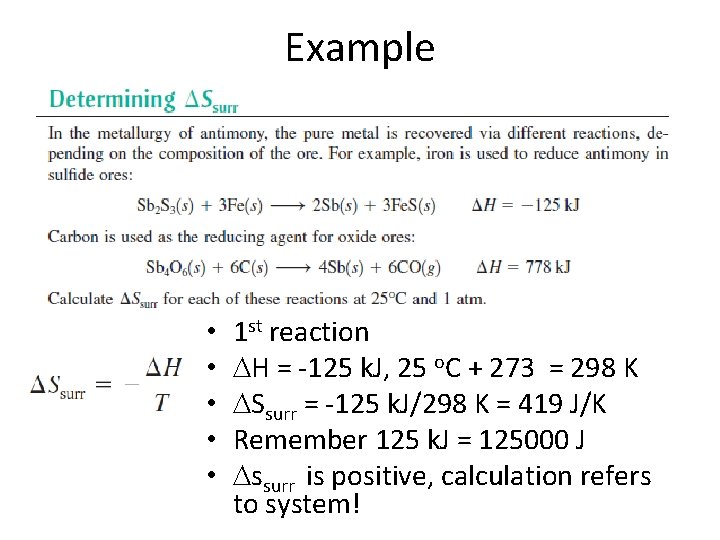
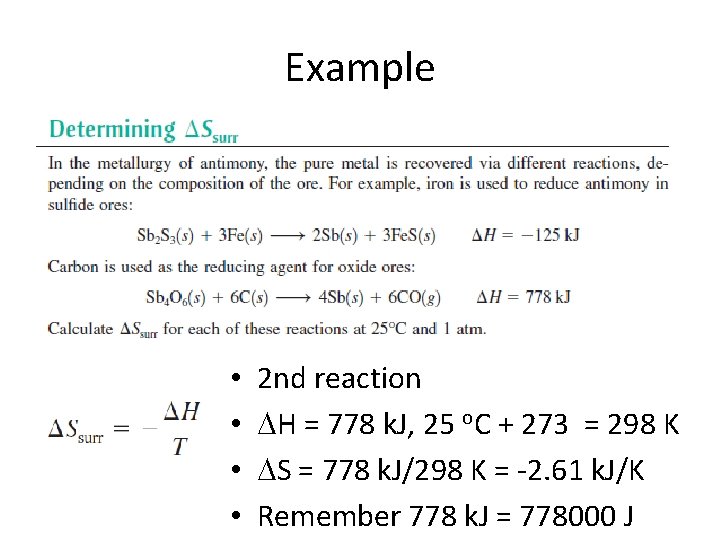
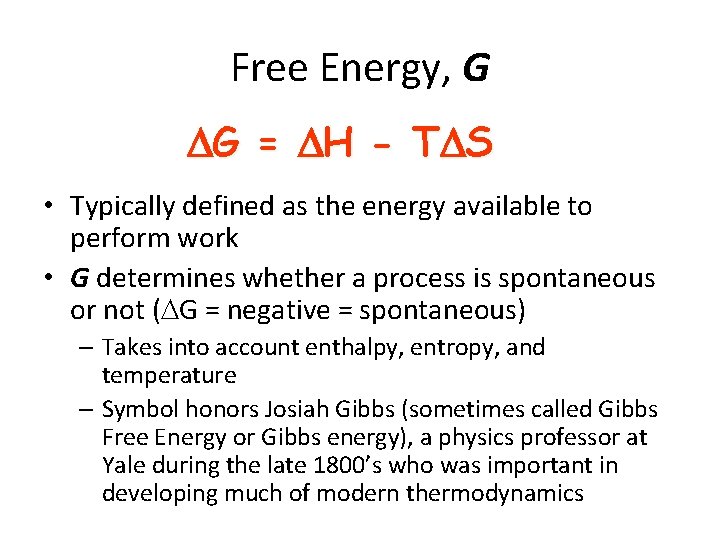
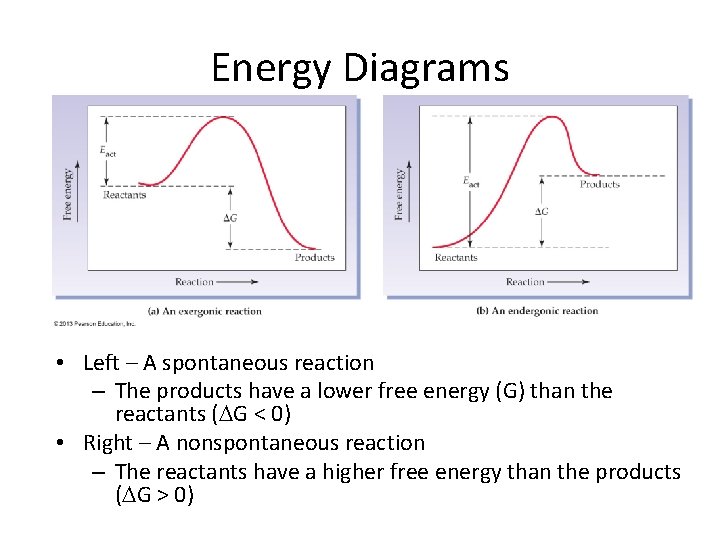
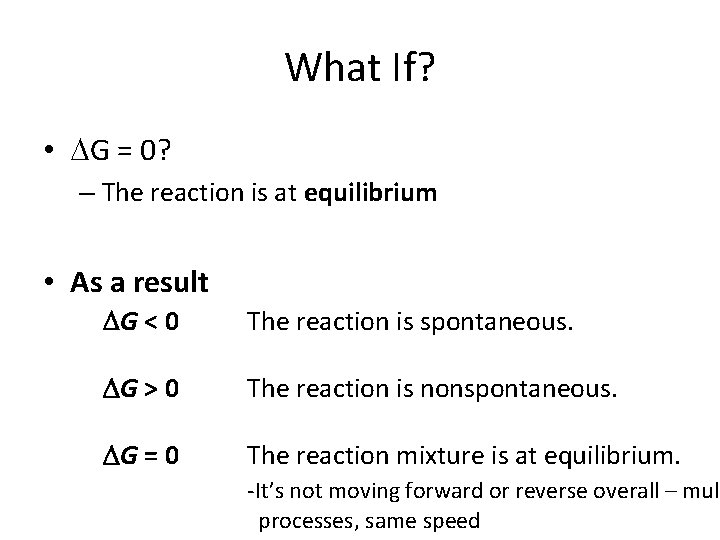
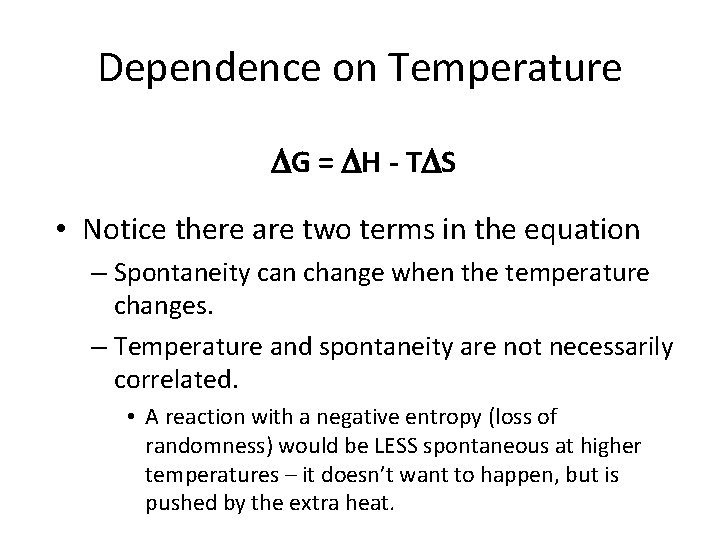
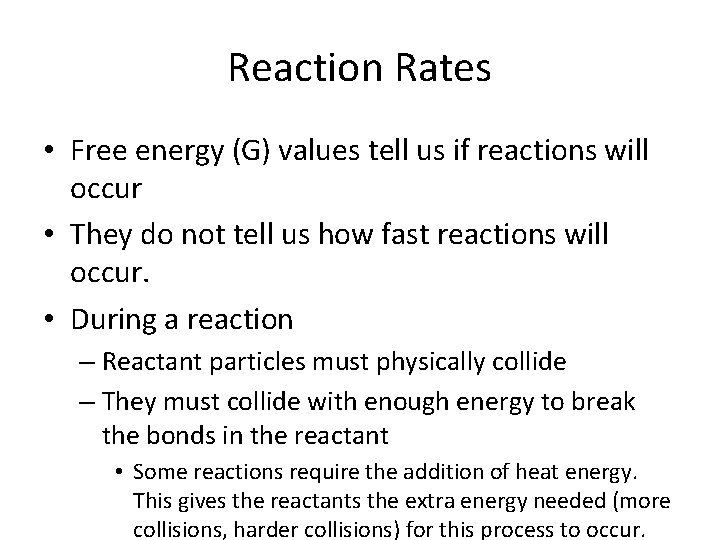
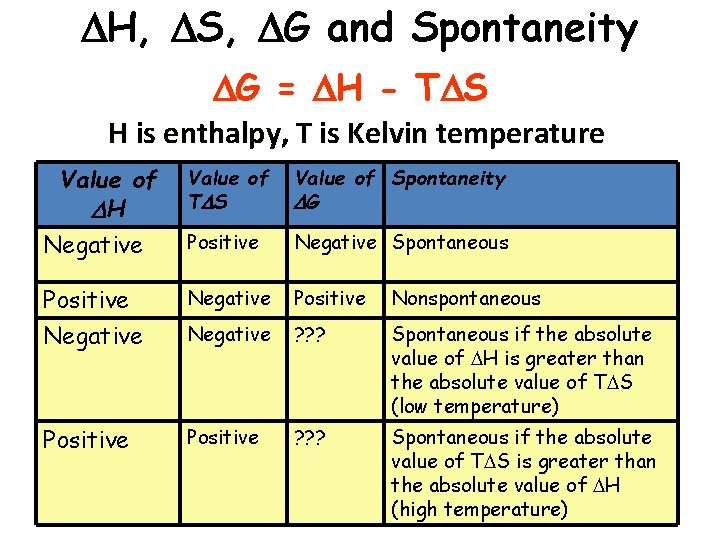
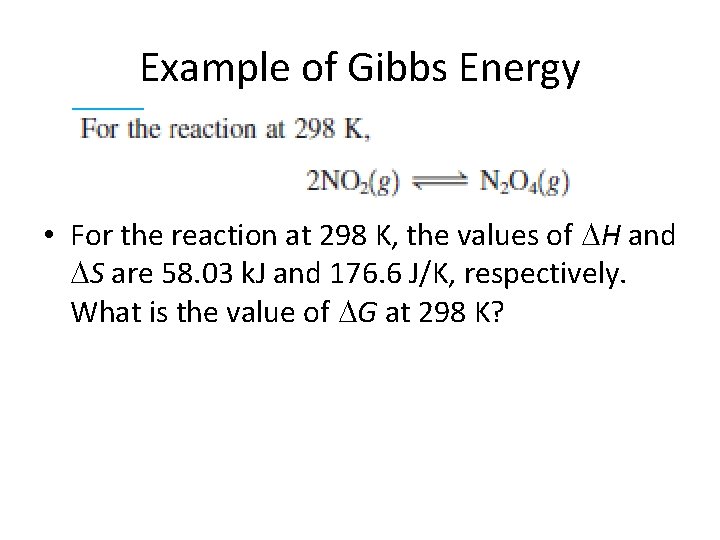
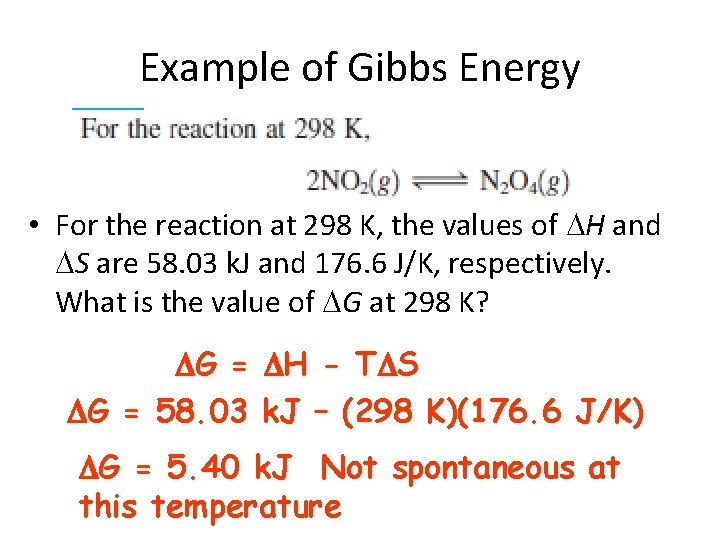
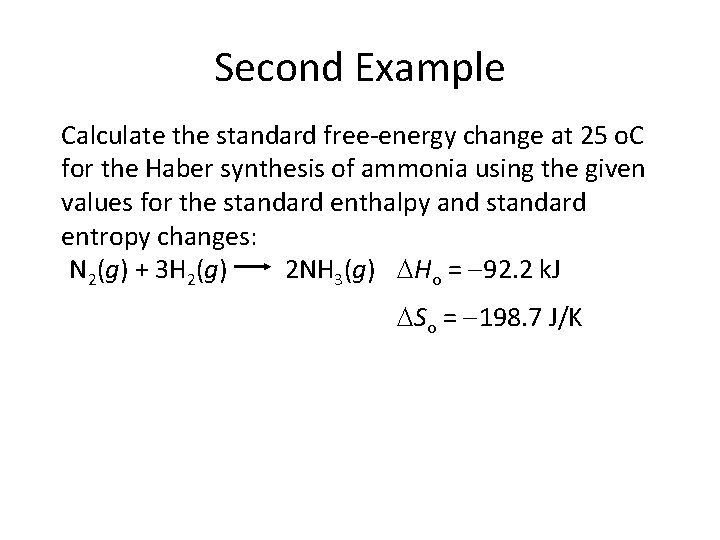
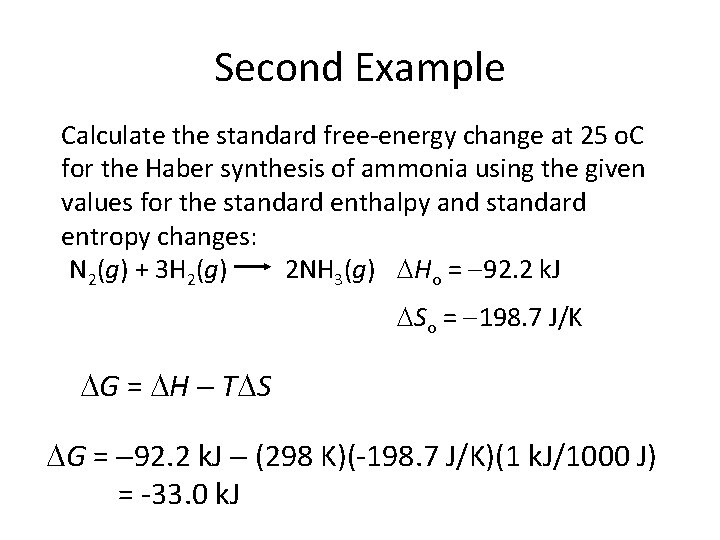
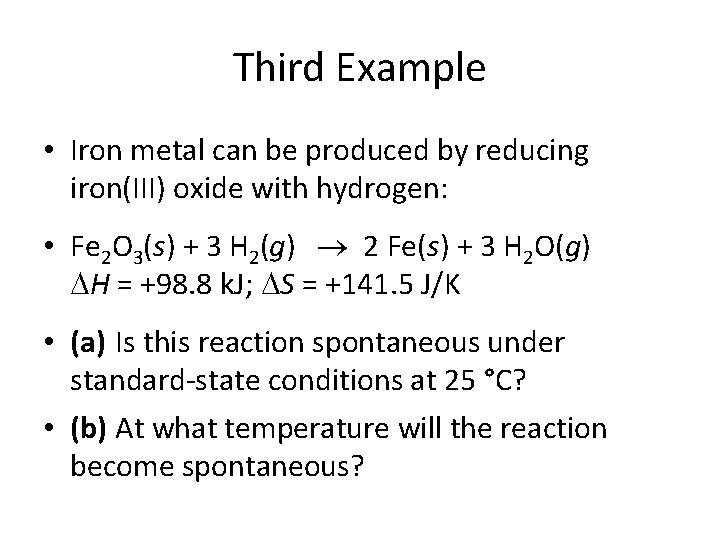
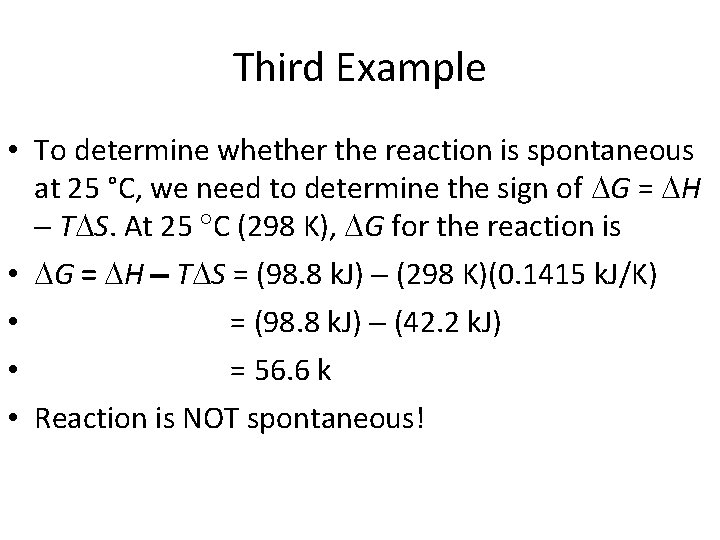
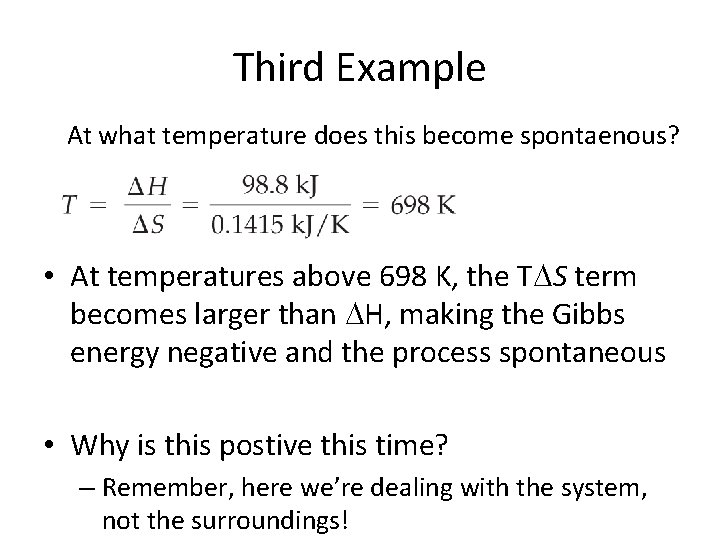
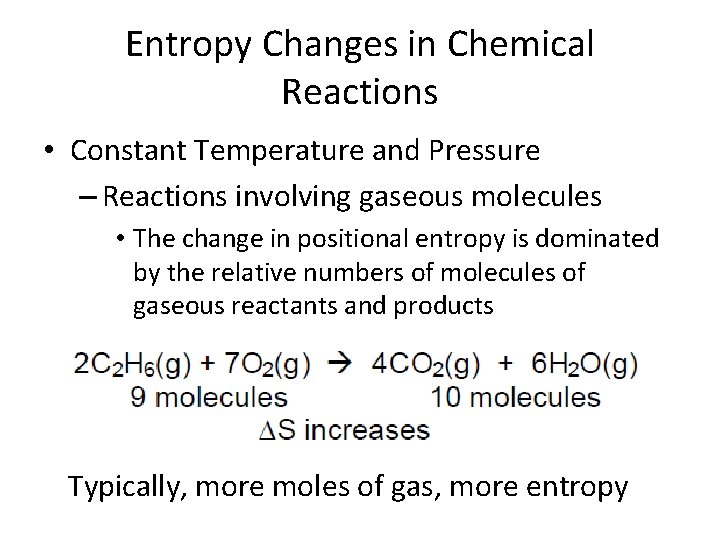
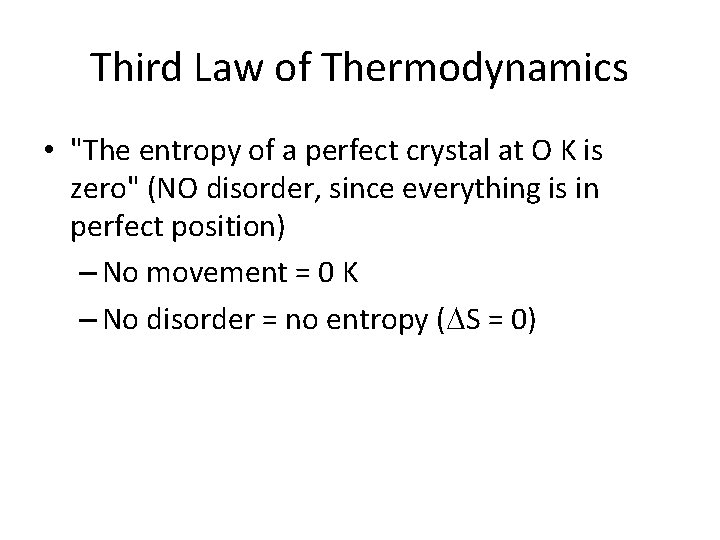
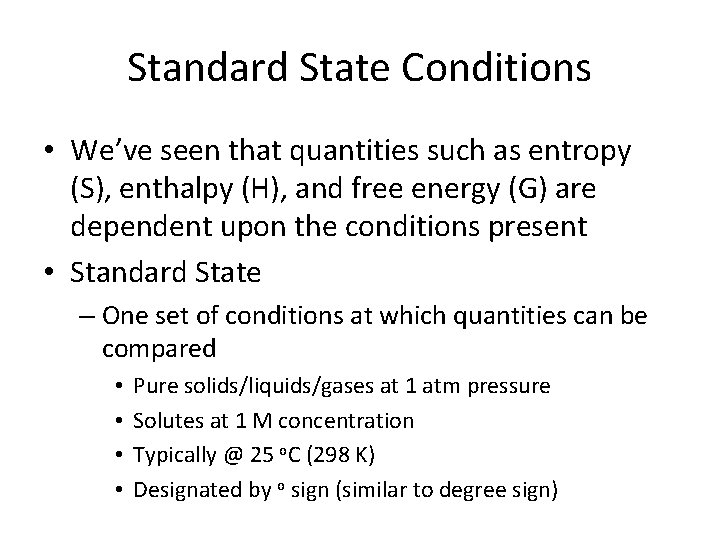
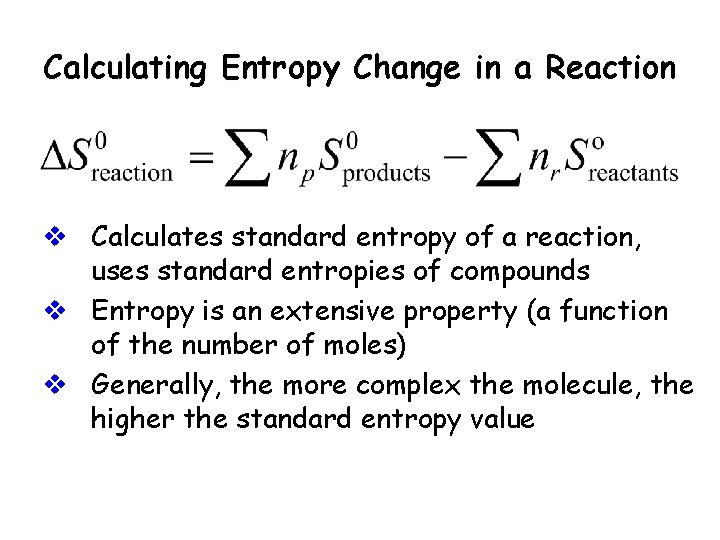
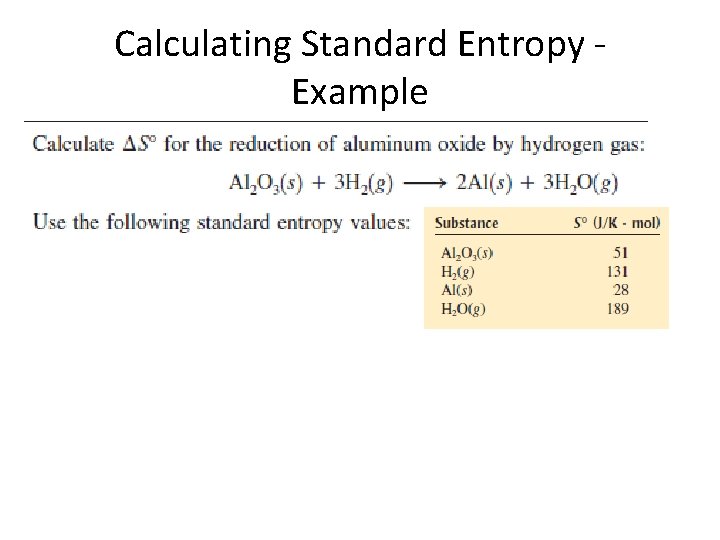
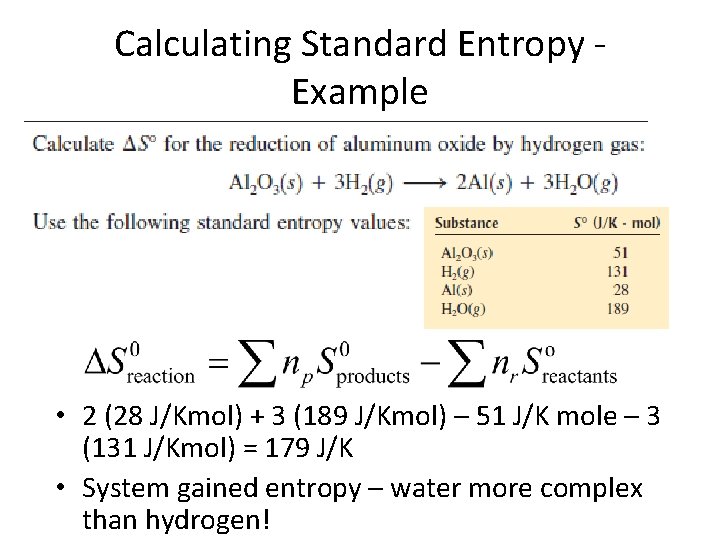
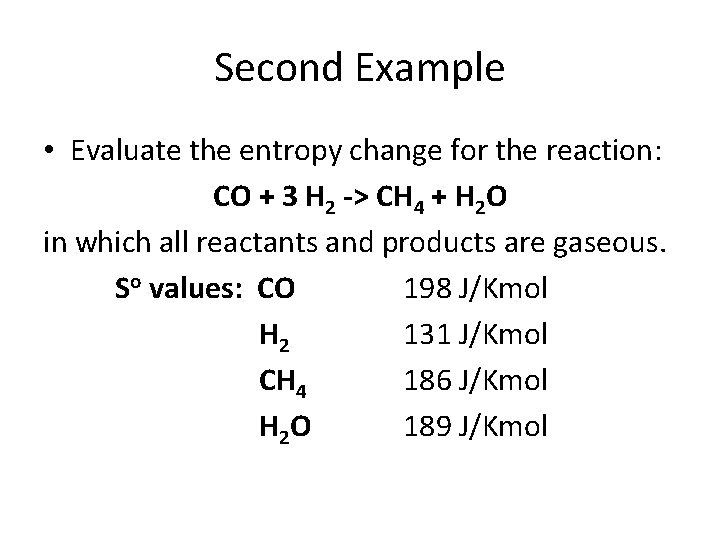
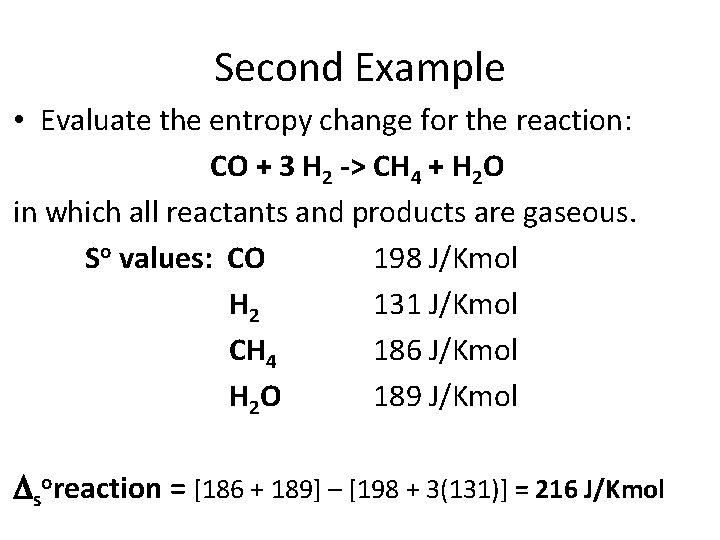
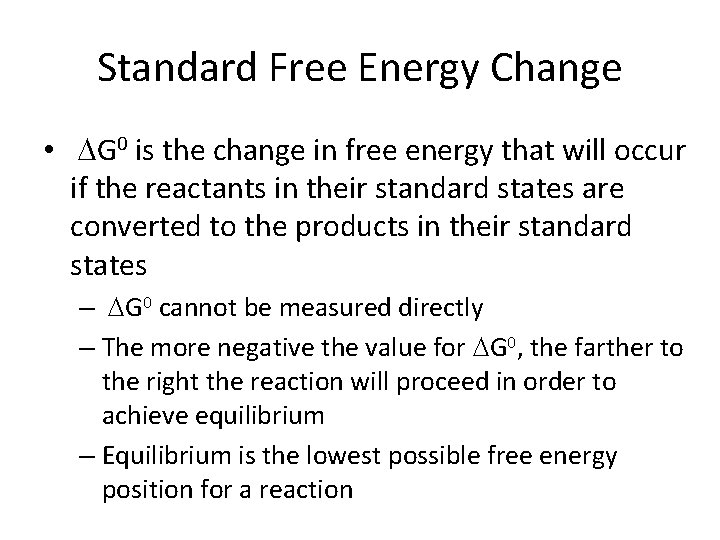
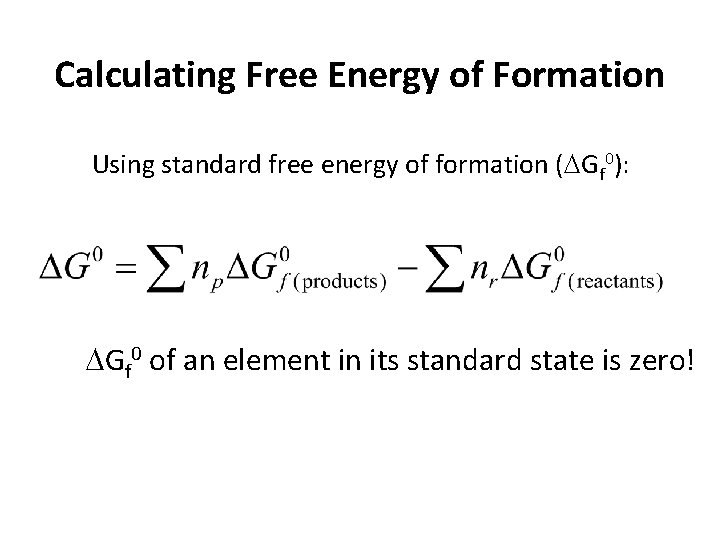
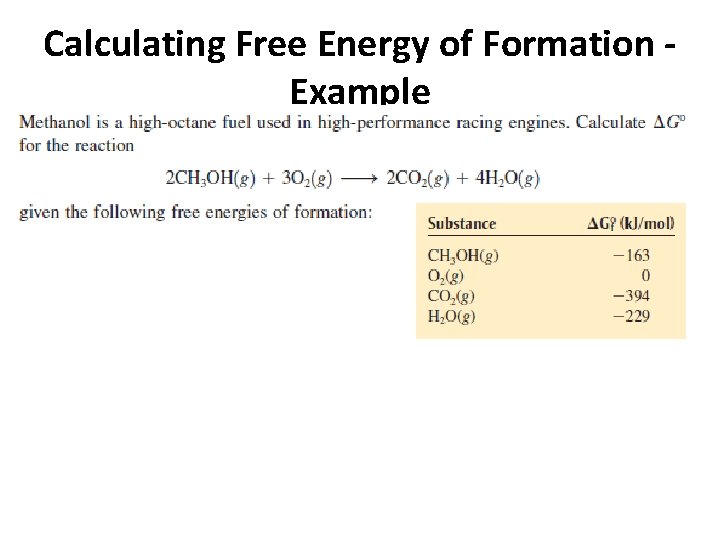
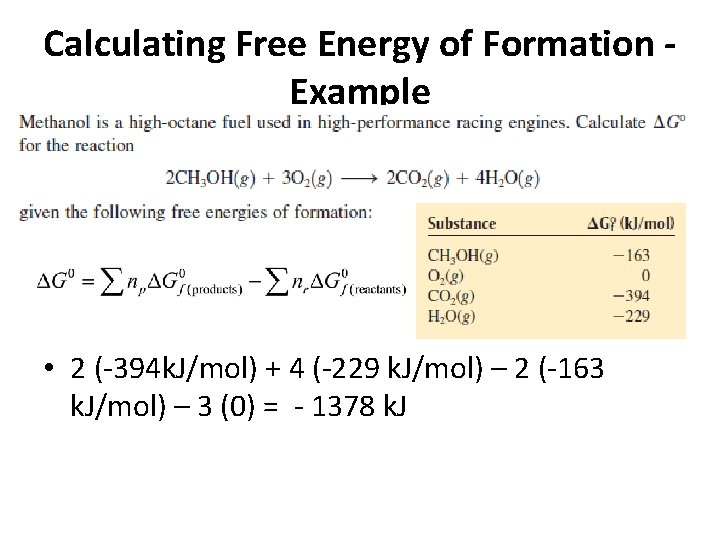
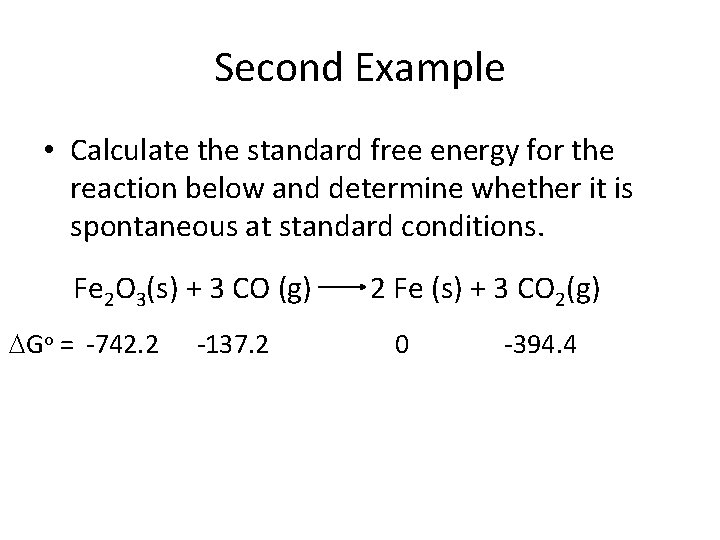
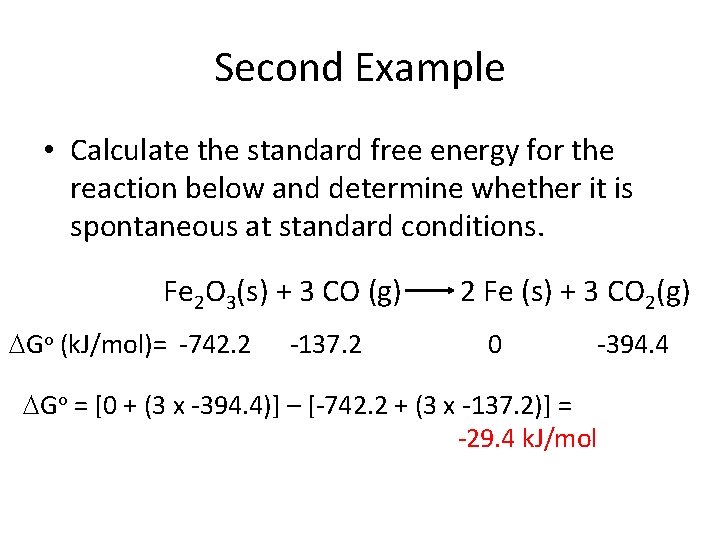
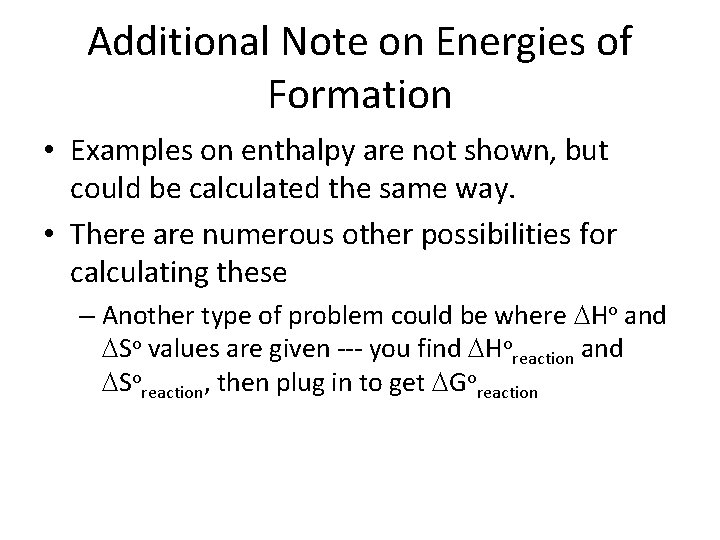
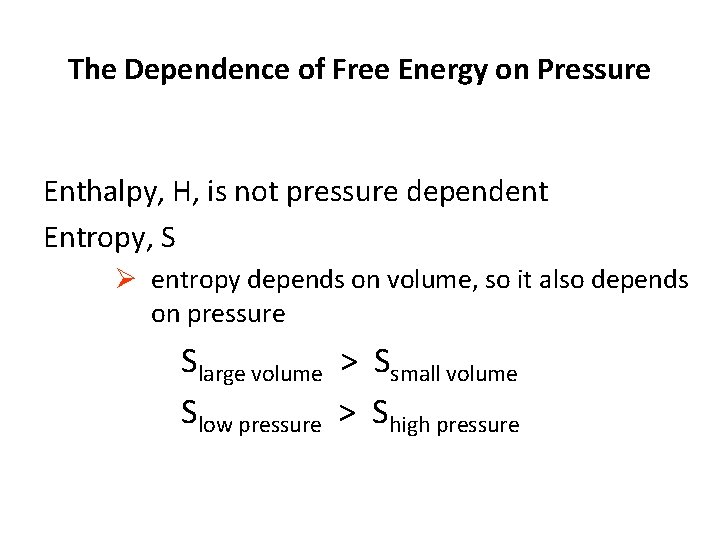
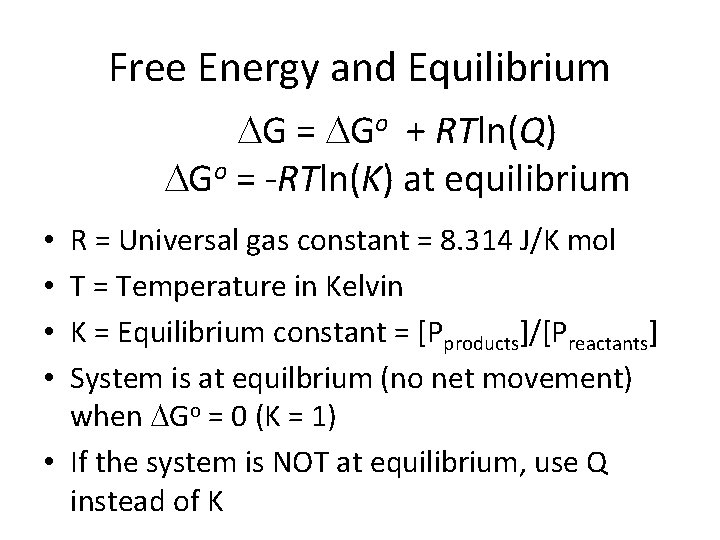
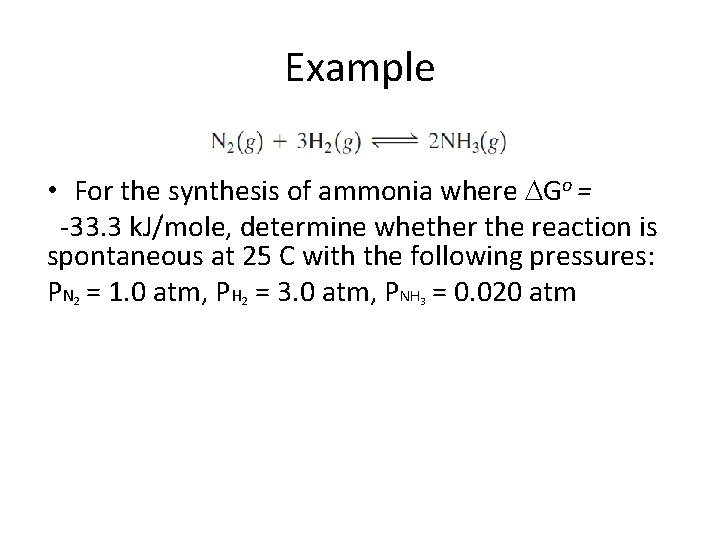
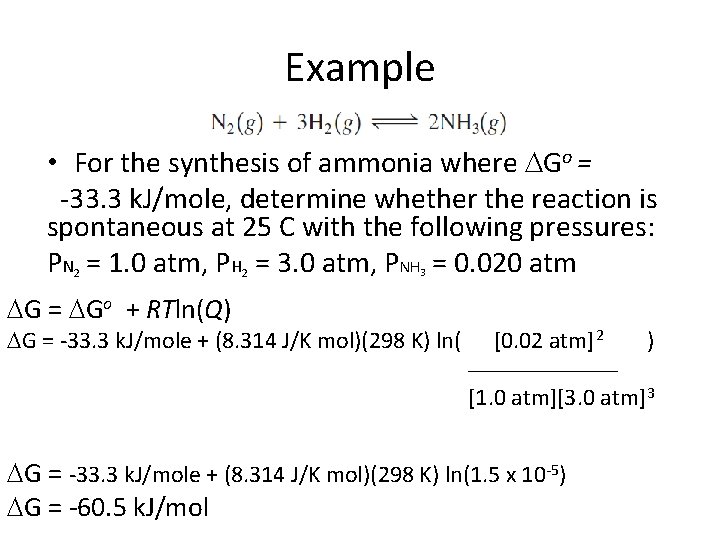
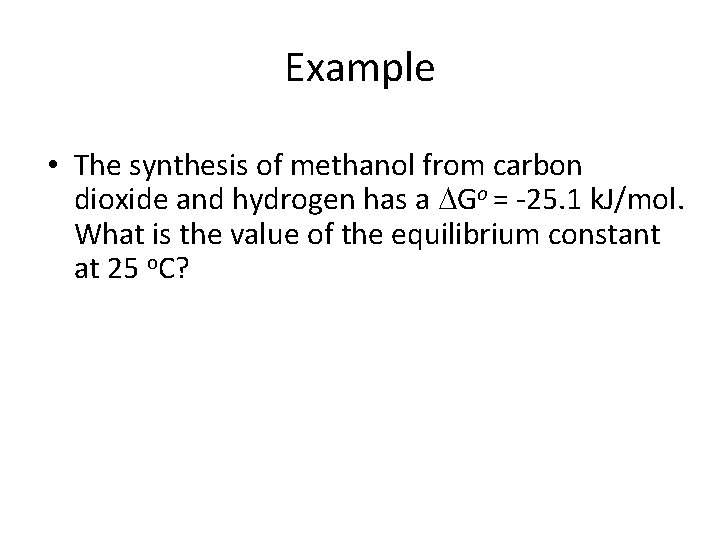
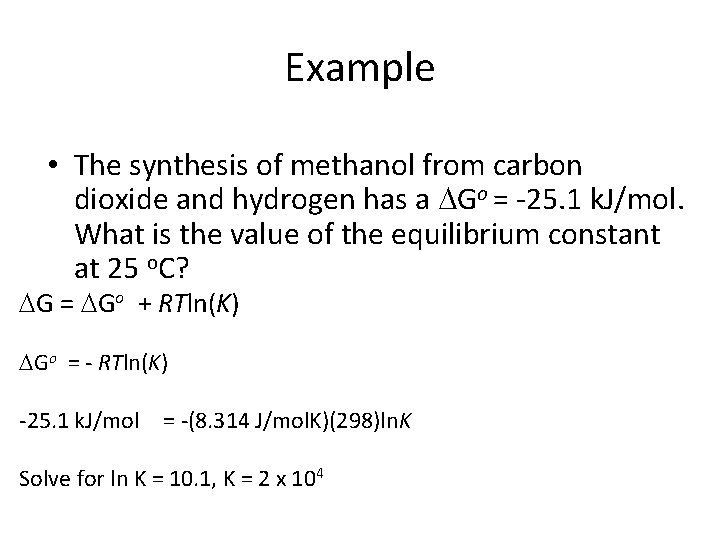
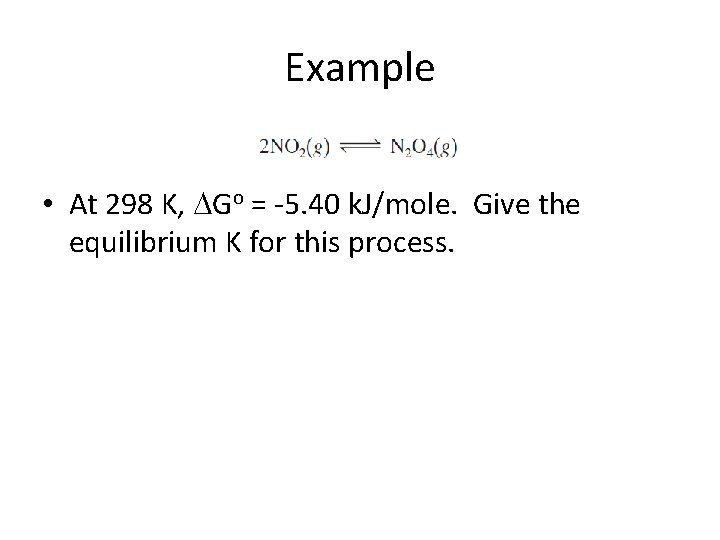
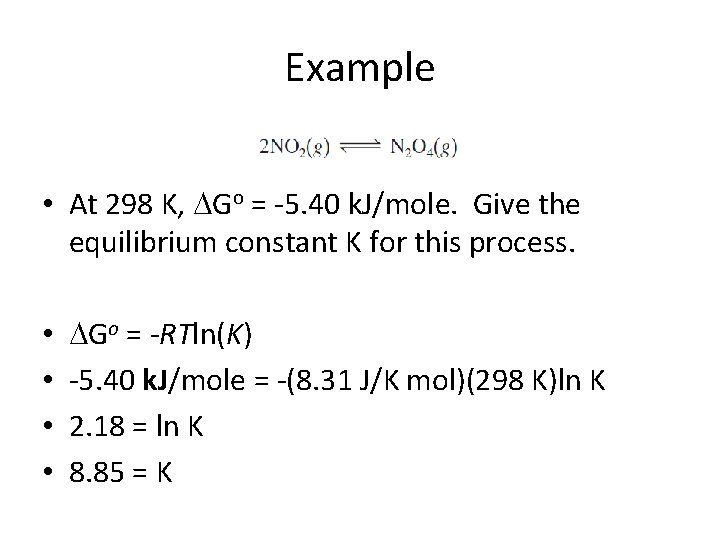
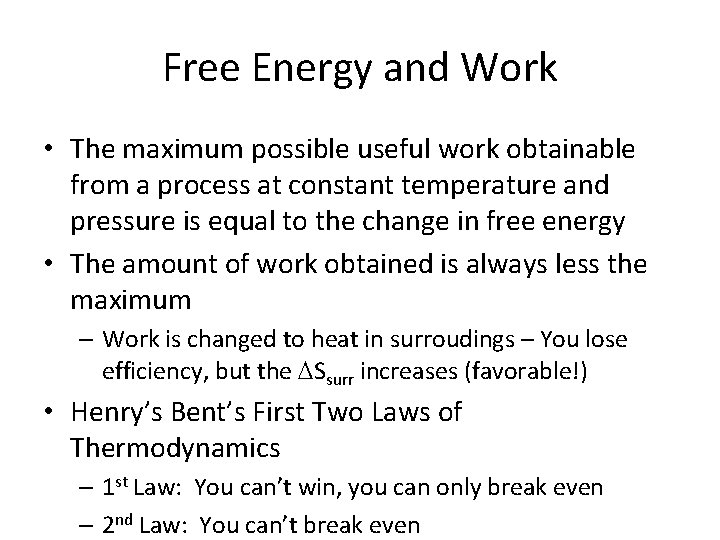
- Slides: 50
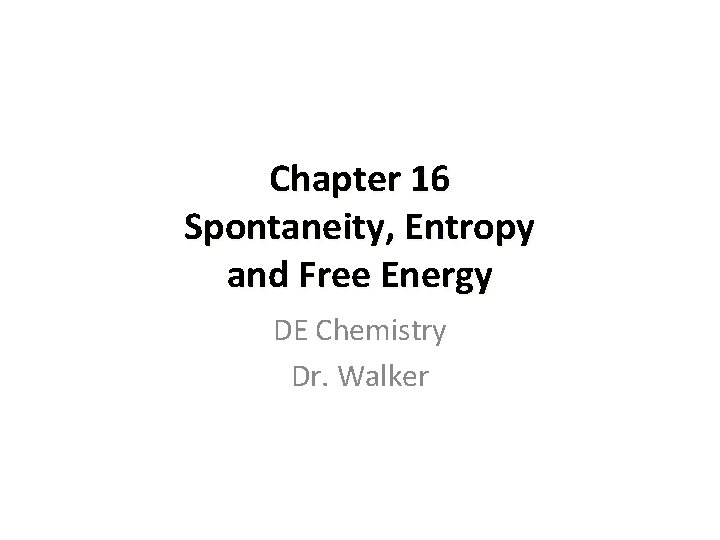
Chapter 16 Spontaneity, Entropy and Free Energy DE Chemistry Dr. Walker
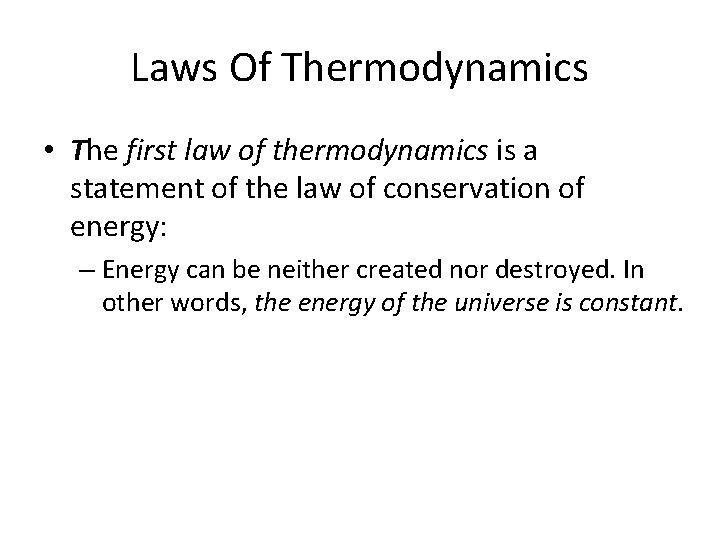
Laws Of Thermodynamics • The first law of thermodynamics is a statement of the law of conservation of energy: – Energy can be neither created nor destroyed. In other words, the energy of the universe is constant.
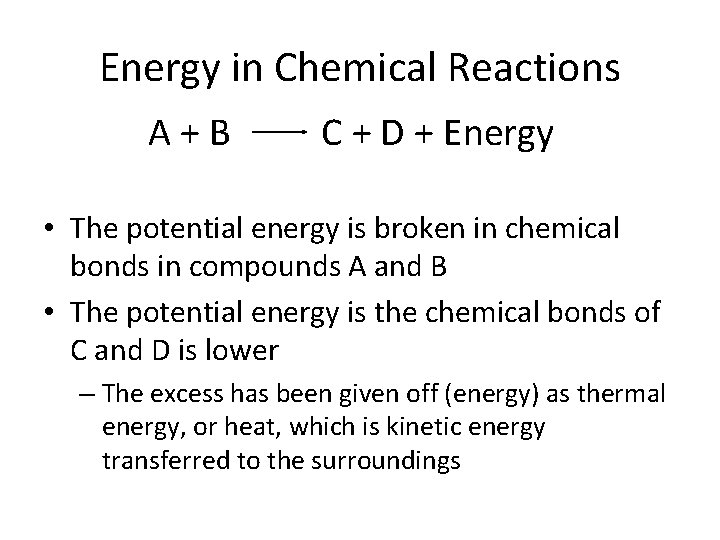
Energy in Chemical Reactions A+B C + D + Energy • The potential energy is broken in chemical bonds in compounds A and B • The potential energy is the chemical bonds of C and D is lower – The excess has been given off (energy) as thermal energy, or heat, which is kinetic energy transferred to the surroundings
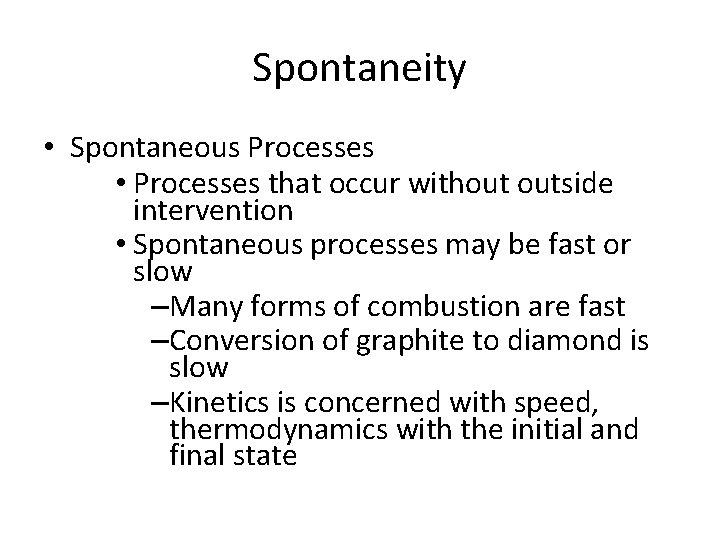
Spontaneity • Spontaneous Processes • Processes that occur without outside intervention • Spontaneous processes may be fast or slow –Many forms of combustion are fast –Conversion of graphite to diamond is slow –Kinetics is concerned with speed, thermodynamics with the initial and final state
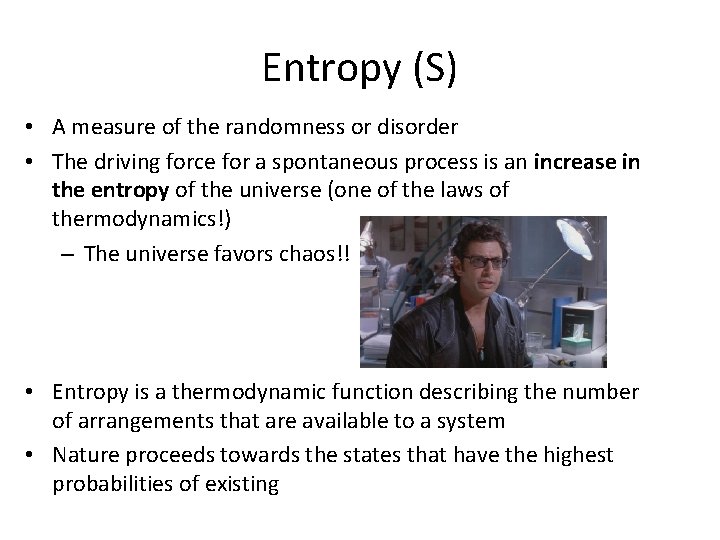
Entropy (S) • A measure of the randomness or disorder • The driving force for a spontaneous process is an increase in the entropy of the universe (one of the laws of thermodynamics!) – The universe favors chaos!! • Entropy is a thermodynamic function describing the number of arrangements that are available to a system • Nature proceeds towards the states that have the highest probabilities of existing
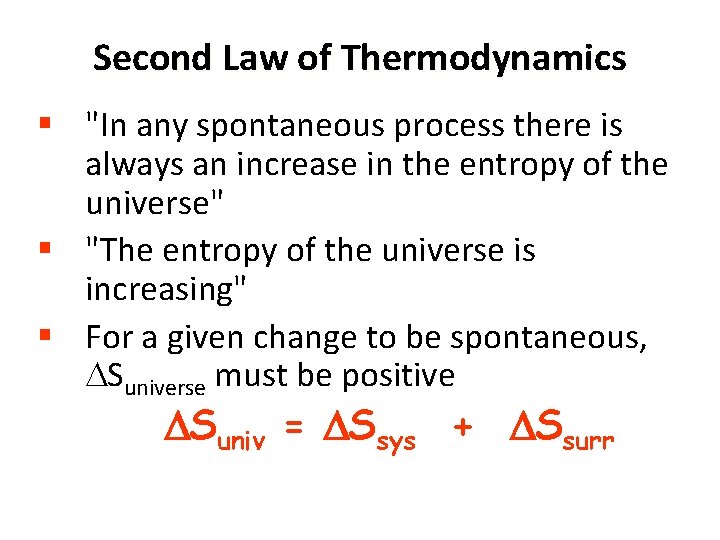
Second Law of Thermodynamics § "In any spontaneous process there is always an increase in the entropy of the universe" § "The entropy of the universe is increasing" § For a given change to be spontaneous, Suniverse must be positive Suniv = Ssys + Ssurr
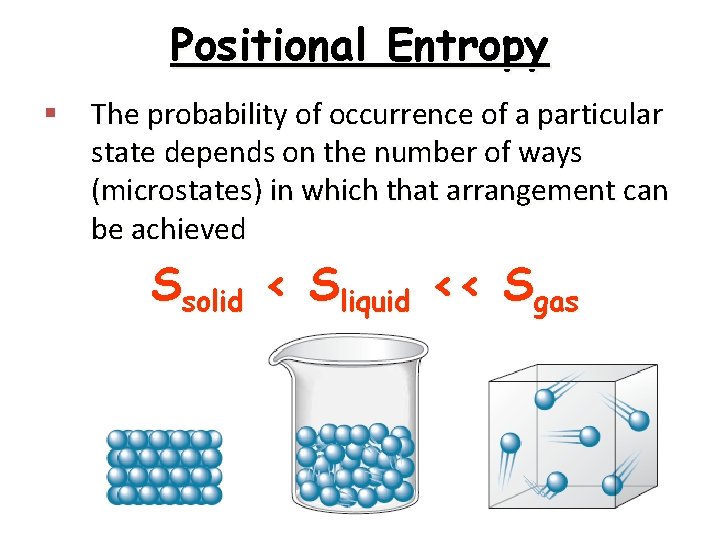
Positional Entropy § The probability of occurrence of a particular state depends on the number of ways (microstates) in which that arrangement can be achieved Ssolid < Sliquid << Sgas
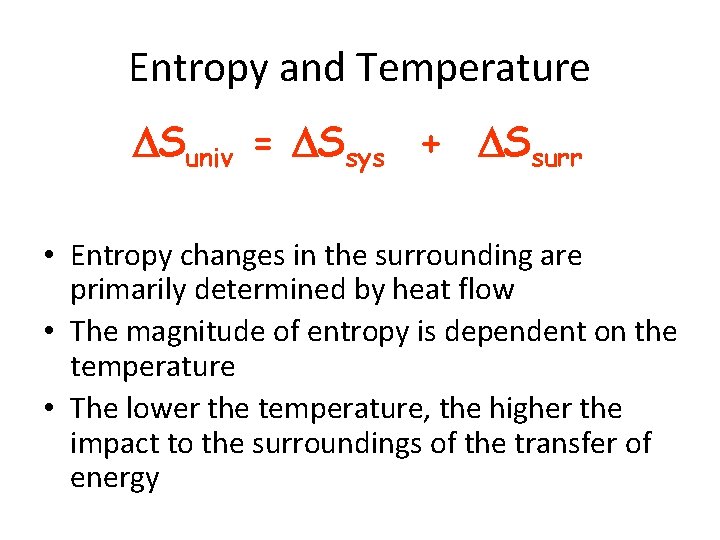
Entropy and Temperature Suniv = Ssys + Ssurr • Entropy changes in the surrounding are primarily determined by heat flow • The magnitude of entropy is dependent on the temperature • The lower the temperature, the higher the impact to the surroundings of the transfer of energy
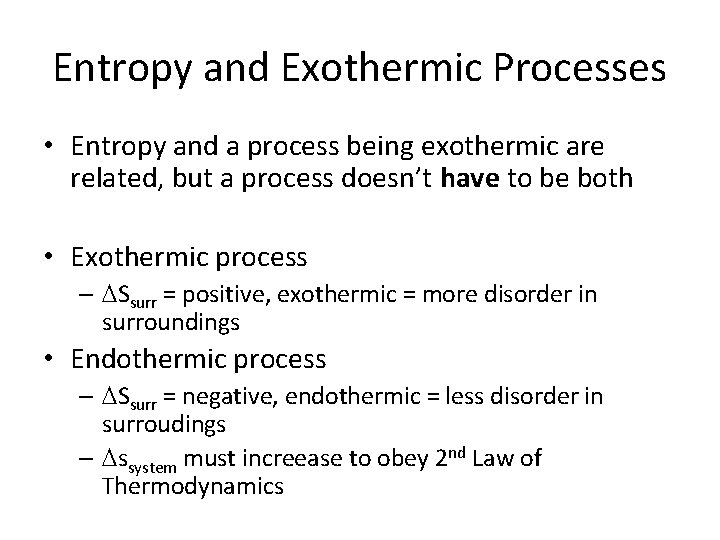
Entropy and Exothermic Processes • Entropy and a process being exothermic are related, but a process doesn’t have to be both • Exothermic process – Ssurr = positive, exothermic = more disorder in surroundings • Endothermic process – Ssurr = negative, endothermic = less disorder in surroudings – ssystem must increease to obey 2 nd Law of Thermodynamics
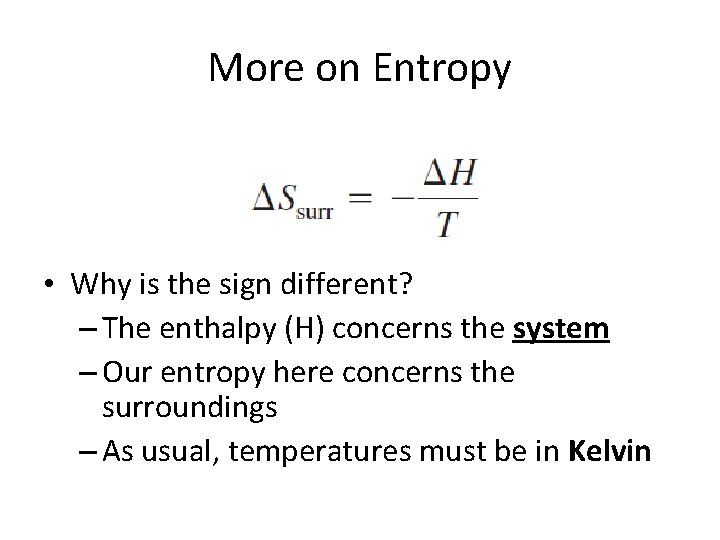
More on Entropy • Why is the sign different? – The enthalpy (H) concerns the system – Our entropy here concerns the surroundings – As usual, temperatures must be in Kelvin
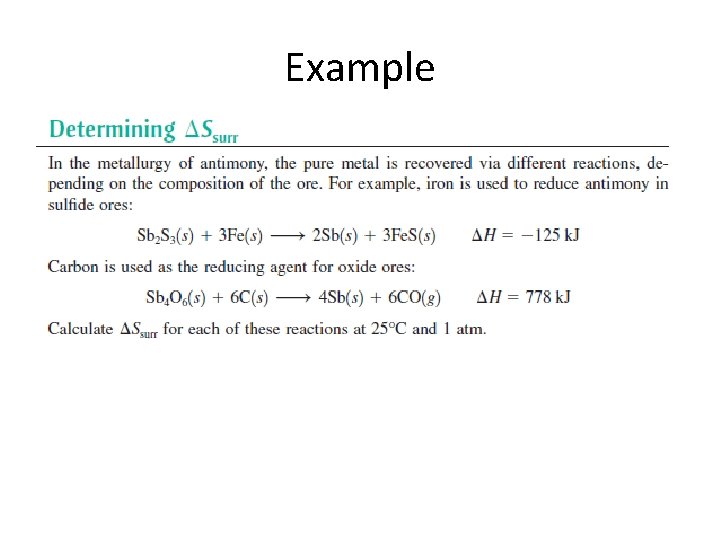
Example
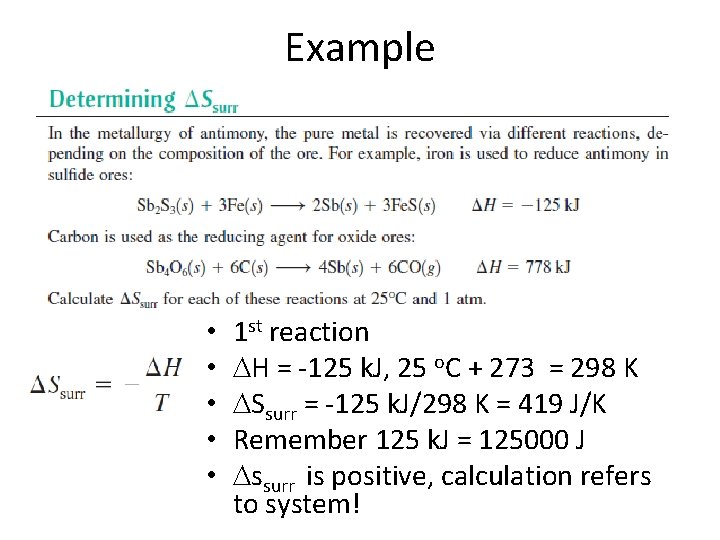
Example • • • 1 st reaction H = -125 k. J, 25 o. C + 273 = 298 K Ssurr = -125 k. J/298 K = 419 J/K Remember 125 k. J = 125000 J ssurr is positive, calculation refers to system!
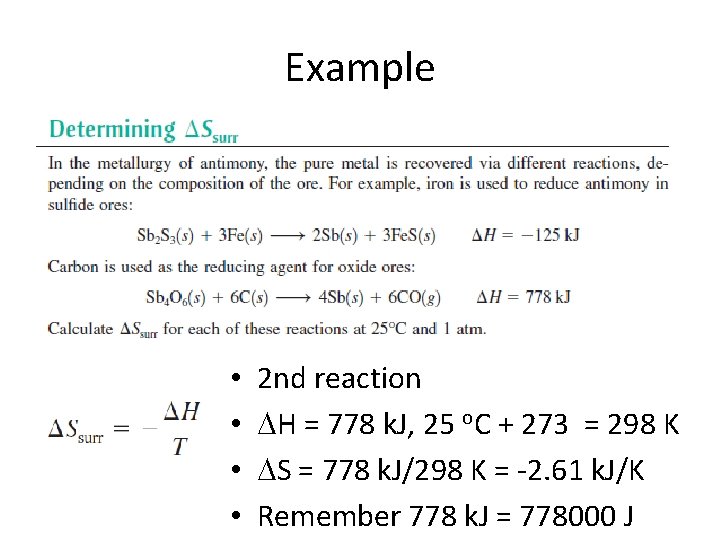
Example • • 2 nd reaction H = 778 k. J, 25 o. C + 273 = 298 K S = 778 k. J/298 K = -2. 61 k. J/K Remember 778 k. J = 778000 J
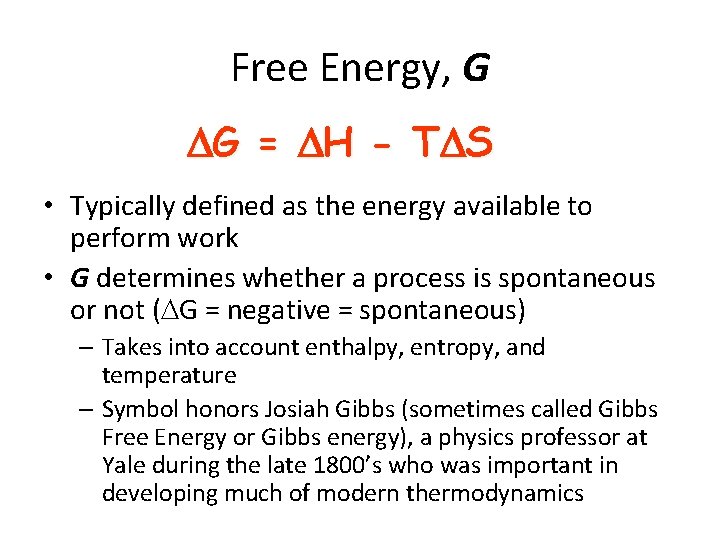
Free Energy, G G = H - T S • Typically defined as the energy available to perform work • G determines whether a process is spontaneous or not ( G = negative = spontaneous) – Takes into account enthalpy, entropy, and temperature – Symbol honors Josiah Gibbs (sometimes called Gibbs Free Energy or Gibbs energy), a physics professor at Yale during the late 1800’s who was important in developing much of modern thermodynamics
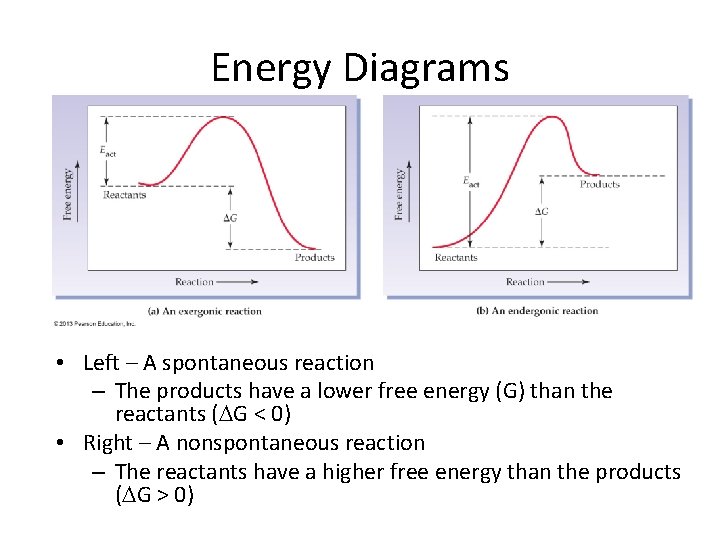
Energy Diagrams • Left – A spontaneous reaction – The products have a lower free energy (G) than the reactants ( G < 0) • Right – A nonspontaneous reaction – The reactants have a higher free energy than the products ( G > 0)
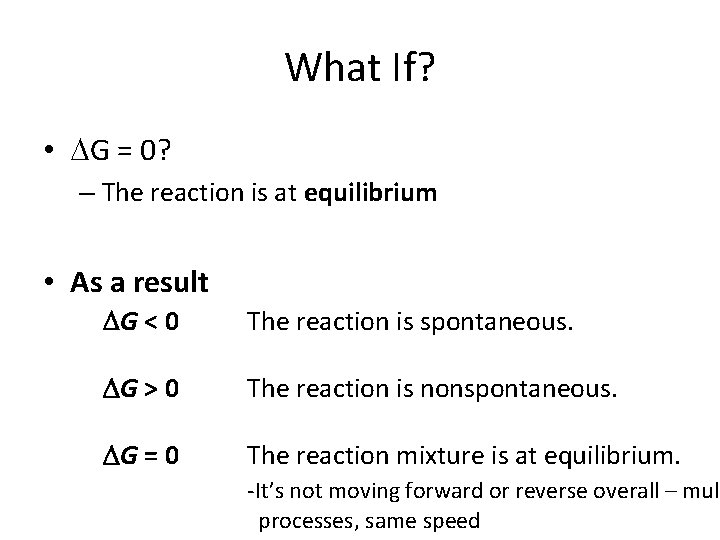
What If? • G = 0? – The reaction is at equilibrium • As a result G < 0 The reaction is spontaneous. G > 0 The reaction is nonspontaneous. G = 0 The reaction mixture is at equilibrium. -It’s not moving forward or reverse overall – mult processes, same speed
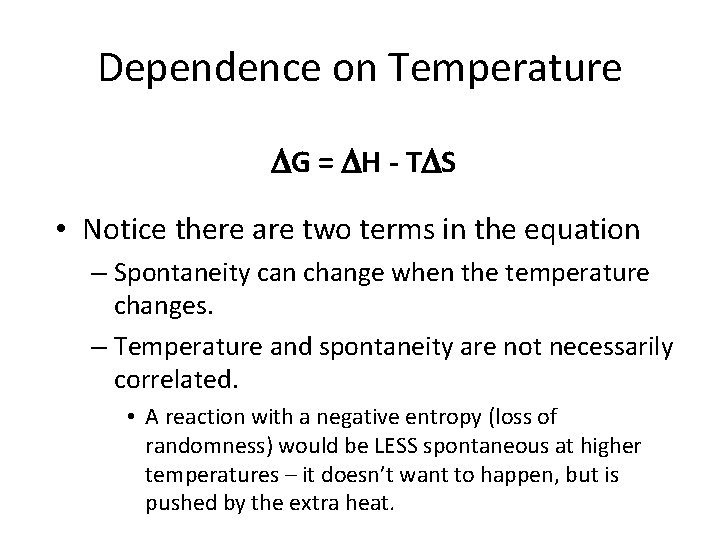
Dependence on Temperature G = H - T S • Notice there are two terms in the equation – Spontaneity can change when the temperature changes. – Temperature and spontaneity are not necessarily correlated. • A reaction with a negative entropy (loss of randomness) would be LESS spontaneous at higher temperatures – it doesn’t want to happen, but is pushed by the extra heat.
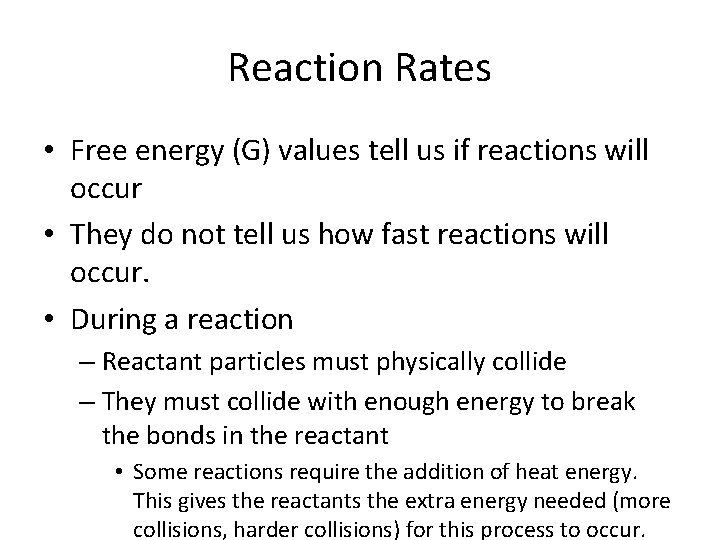
Reaction Rates • Free energy (G) values tell us if reactions will occur • They do not tell us how fast reactions will occur. • During a reaction – Reactant particles must physically collide – They must collide with enough energy to break the bonds in the reactant • Some reactions require the addition of heat energy. This gives the reactants the extra energy needed (more collisions, harder collisions) for this process to occur.
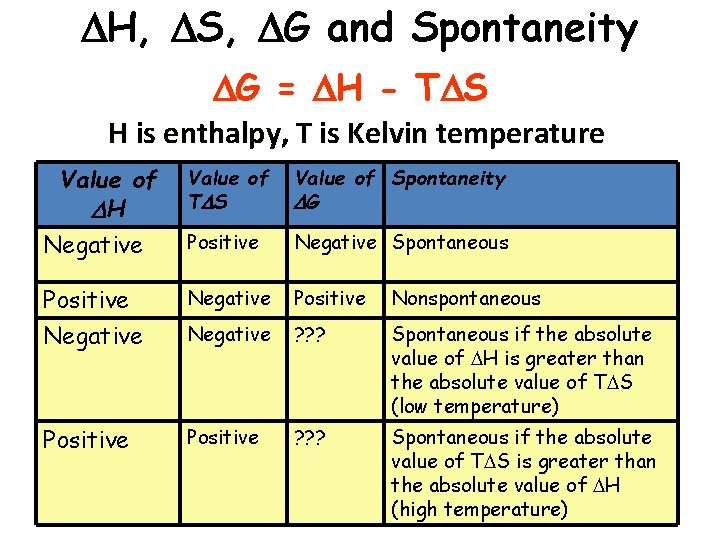
H, S, G and Spontaneity G = H - T S H is enthalpy, T is Kelvin temperature Value of H Negative Value of T S Value of Spontaneity G Positive Negative Spontaneous Positive Negative Positive Nonspontaneous Negative ? ? ? Spontaneous if the absolute value of H is greater than the absolute value of T S (low temperature) Positive ? ? ? Spontaneous if the absolute value of T S is greater than the absolute value of H (high temperature)
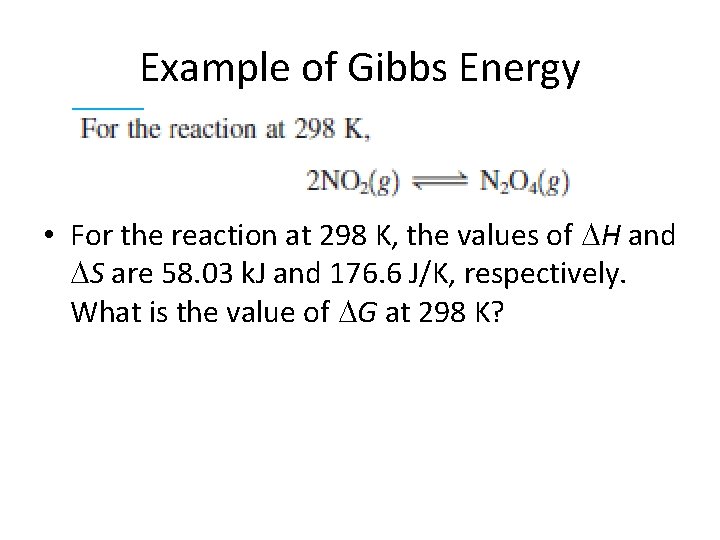
Example of Gibbs Energy • For the reaction at 298 K, the values of H and S are 58. 03 k. J and 176. 6 J/K, respectively. What is the value of G at 298 K?
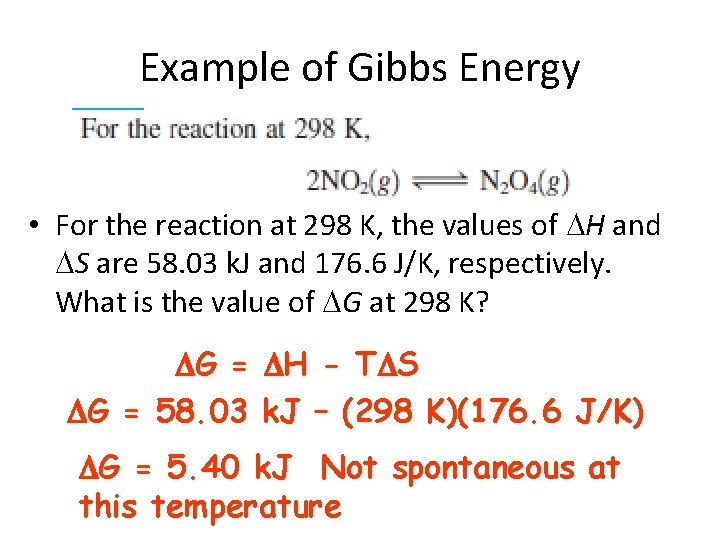
Example of Gibbs Energy • For the reaction at 298 K, the values of H and S are 58. 03 k. J and 176. 6 J/K, respectively. What is the value of G at 298 K? G = H - T S G = 58. 03 k. J – (298 K)(176. 6 J/K) G = 5. 40 k. J Not spontaneous at this temperature
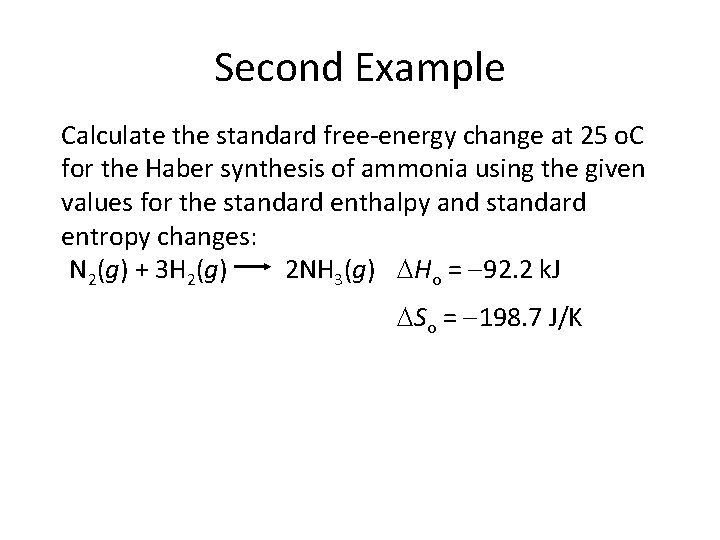
Second Example Calculate the standard free-energy change at 25 o. C for the Haber synthesis of ammonia using the given values for the standard enthalpy and standard entropy changes: N 2(g) + 3 H 2(g) 2 NH 3(g) Ho = 92. 2 k. J So = 198. 7 J/K
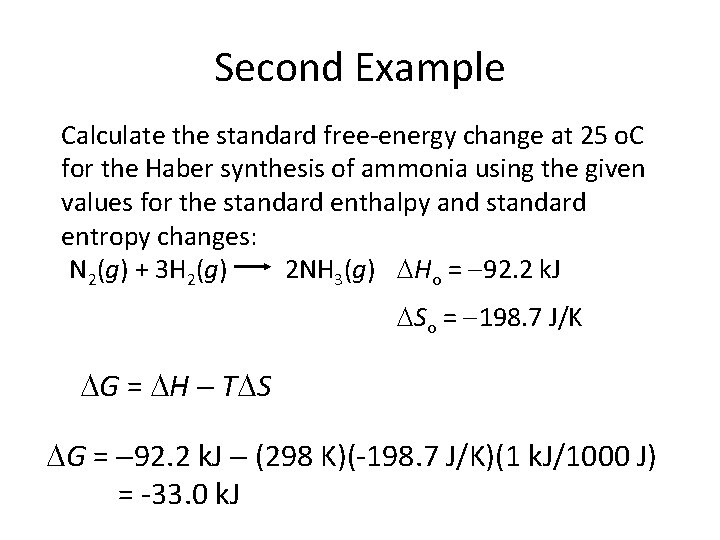
Second Example Calculate the standard free-energy change at 25 o. C for the Haber synthesis of ammonia using the given values for the standard enthalpy and standard entropy changes: N 2(g) + 3 H 2(g) 2 NH 3(g) Ho = 92. 2 k. J So = 198. 7 J/K G = H T S G = 92. 2 k. J (298 K)(-198. 7 J/K)(1 k. J/1000 J) = -33. 0 k. J
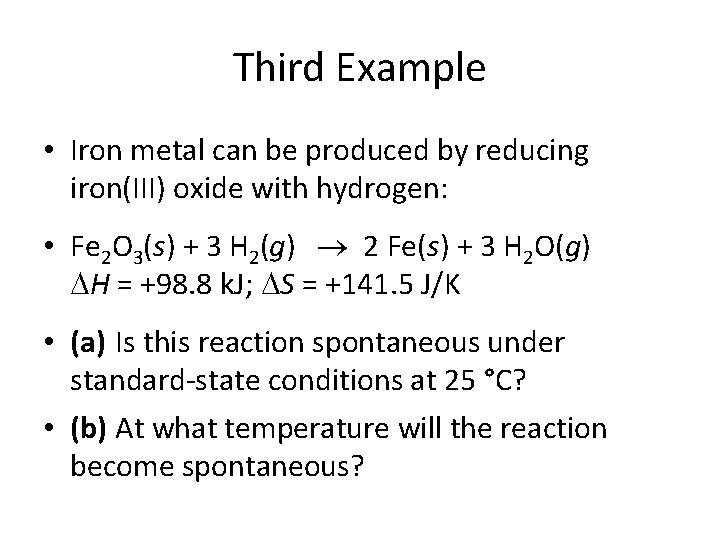
Third Example • Iron metal can be produced by reducing iron(III) oxide with hydrogen: • Fe 2 O 3(s) + 3 H 2(g) 2 Fe(s) + 3 H 2 O(g) H = +98. 8 k. J; S = +141. 5 J/K • (a) Is this reaction spontaneous under standard-state conditions at 25 °C? • (b) At what temperature will the reaction become spontaneous?
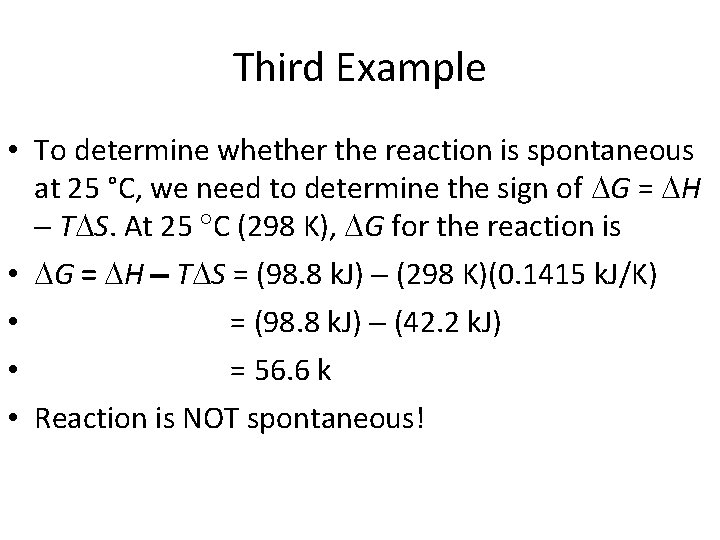
Third Example • To determine whether the reaction is spontaneous at 25 °C, we need to determine the sign of G = H T S. At 25 C (298 K), G for the reaction is • G = H T S = (98. 8 k. J) (298 K)(0. 1415 k. J/K) • = (98. 8 k. J) (42. 2 k. J) • = 56. 6 k • Reaction is NOT spontaneous!
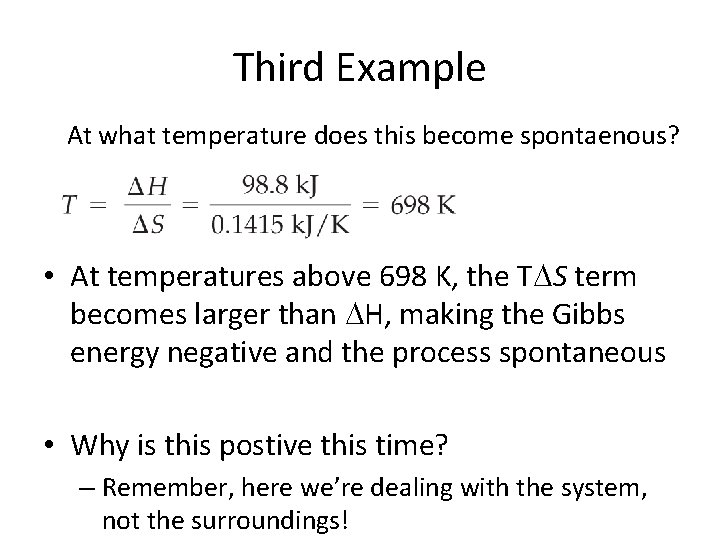
Third Example At what temperature does this become spontaenous? • At temperatures above 698 K, the T S term becomes larger than H, making the Gibbs energy negative and the process spontaneous • Why is this postive this time? – Remember, here we’re dealing with the system, not the surroundings!
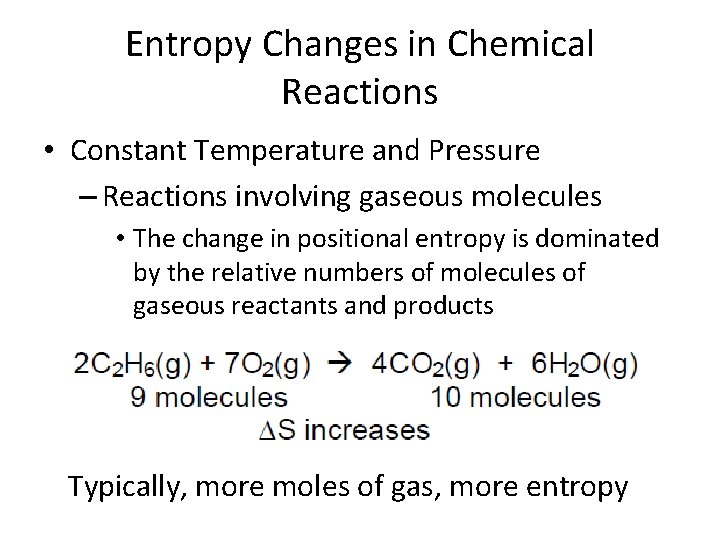
Entropy Changes in Chemical Reactions • Constant Temperature and Pressure – Reactions involving gaseous molecules • The change in positional entropy is dominated by the relative numbers of molecules of gaseous reactants and products Typically, more moles of gas, more entropy
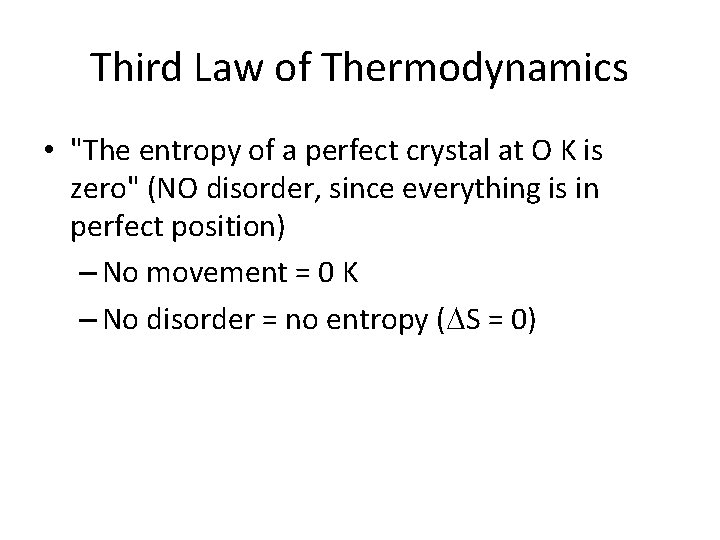
Third Law of Thermodynamics • "The entropy of a perfect crystal at O K is zero" (NO disorder, since everything is in perfect position) – No movement = 0 K – No disorder = no entropy ( S = 0)
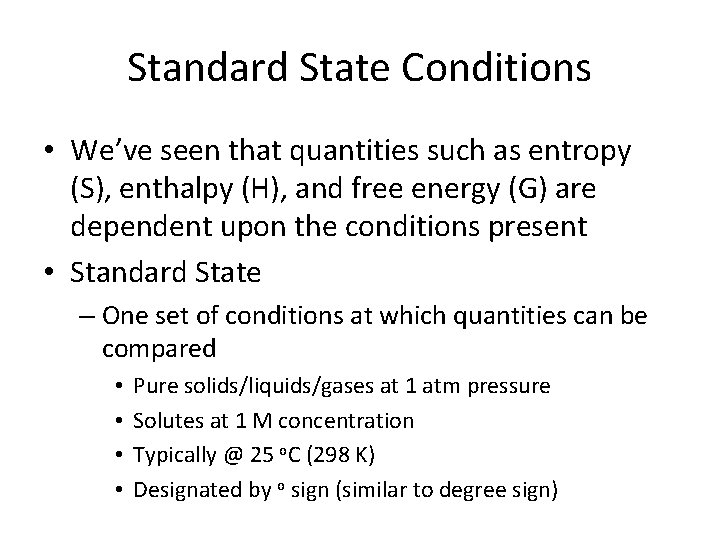
Standard State Conditions • We’ve seen that quantities such as entropy (S), enthalpy (H), and free energy (G) are dependent upon the conditions present • Standard State – One set of conditions at which quantities can be compared • • Pure solids/liquids/gases at 1 atm pressure Solutes at 1 M concentration Typically @ 25 o. C (298 K) Designated by o sign (similar to degree sign)
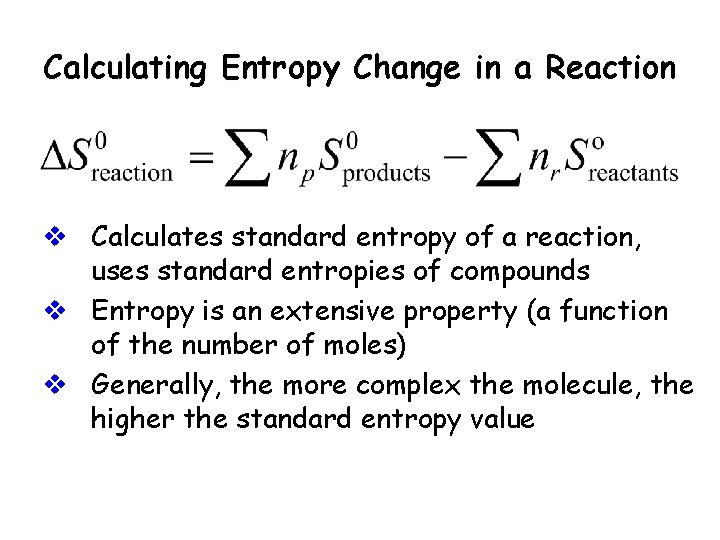
Calculating Entropy Change in a Reaction v Calculates standard entropy of a reaction, uses standard entropies of compounds v Entropy is an extensive property (a function of the number of moles) v Generally, the more complex the molecule, the higher the standard entropy value
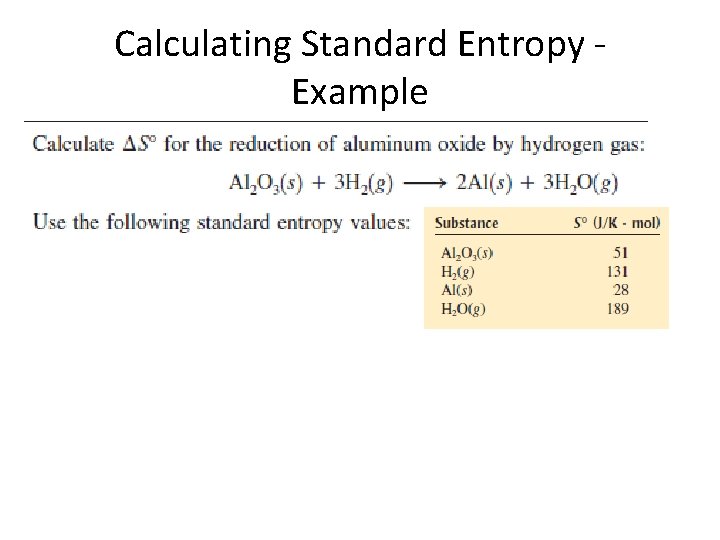
Calculating Standard Entropy Example
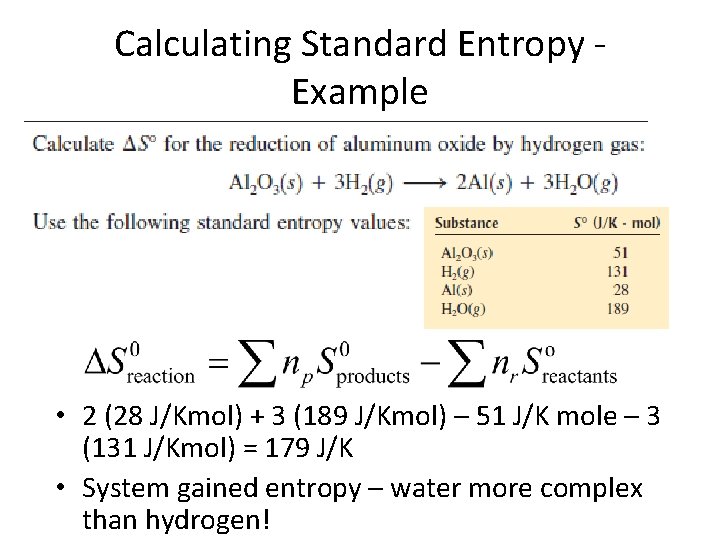
Calculating Standard Entropy Example • 2 (28 J/Kmol) + 3 (189 J/Kmol) – 51 J/K mole – 3 (131 J/Kmol) = 179 J/K • System gained entropy – water more complex than hydrogen!
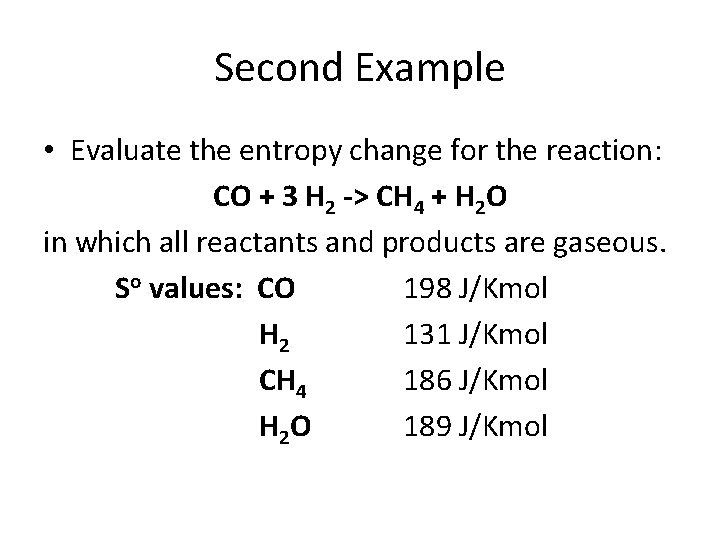
Second Example • Evaluate the entropy change for the reaction: CO + 3 H 2 -> CH 4 + H 2 O in which all reactants and products are gaseous. So values: CO 198 J/Kmol H 2 131 J/Kmol CH 4 186 J/Kmol H 2 O 189 J/Kmol
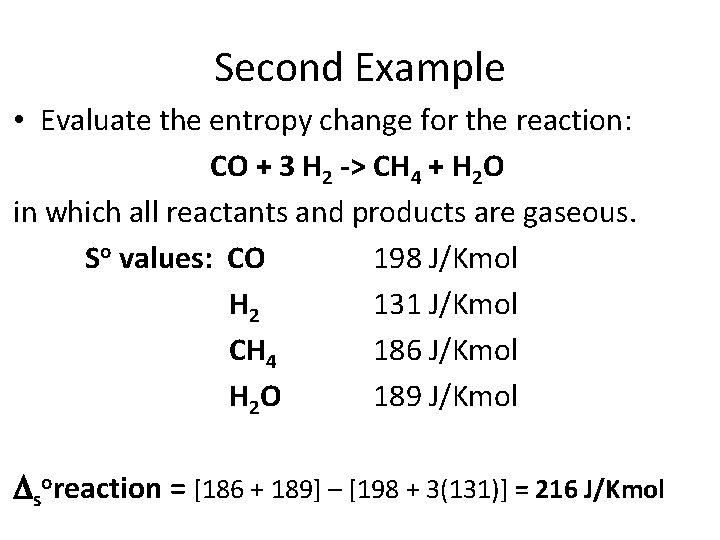
Second Example • Evaluate the entropy change for the reaction: CO + 3 H 2 -> CH 4 + H 2 O in which all reactants and products are gaseous. So values: CO 198 J/Kmol H 2 131 J/Kmol CH 4 186 J/Kmol H 2 O 189 J/Kmol soreaction = [186 + 189] – [198 + 3(131)] = 216 J/Kmol
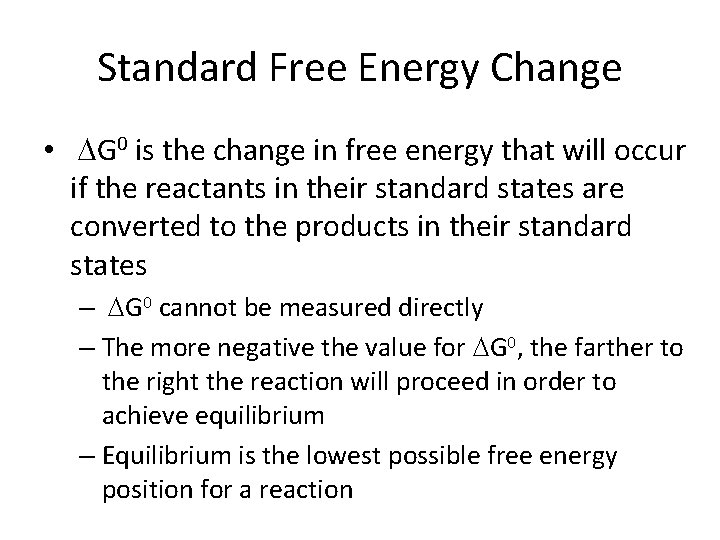
Standard Free Energy Change • G 0 is the change in free energy that will occur if the reactants in their standard states are converted to the products in their standard states – G 0 cannot be measured directly – The more negative the value for G 0, the farther to the right the reaction will proceed in order to achieve equilibrium – Equilibrium is the lowest possible free energy position for a reaction
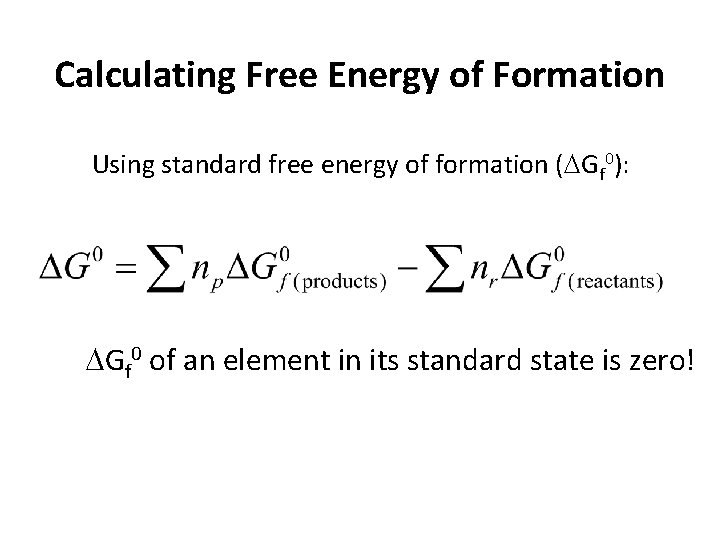
Calculating Free Energy of Formation Using standard free energy of formation ( Gf 0): Gf 0 of an element in its standard state is zero!
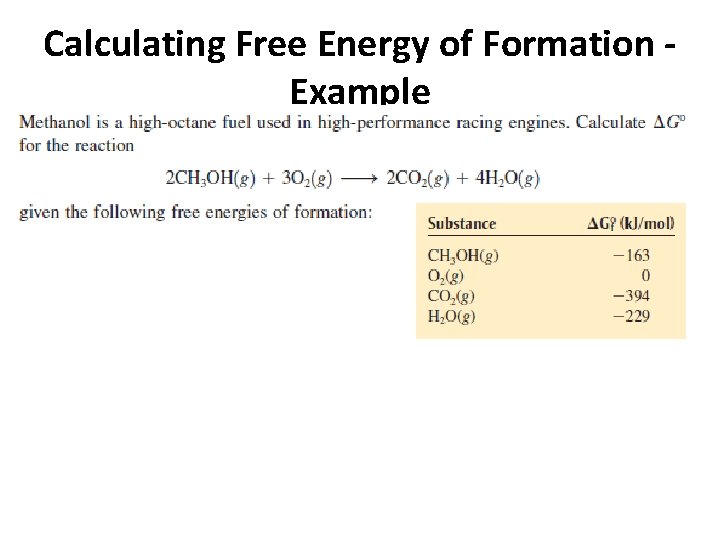
Calculating Free Energy of Formation - Example
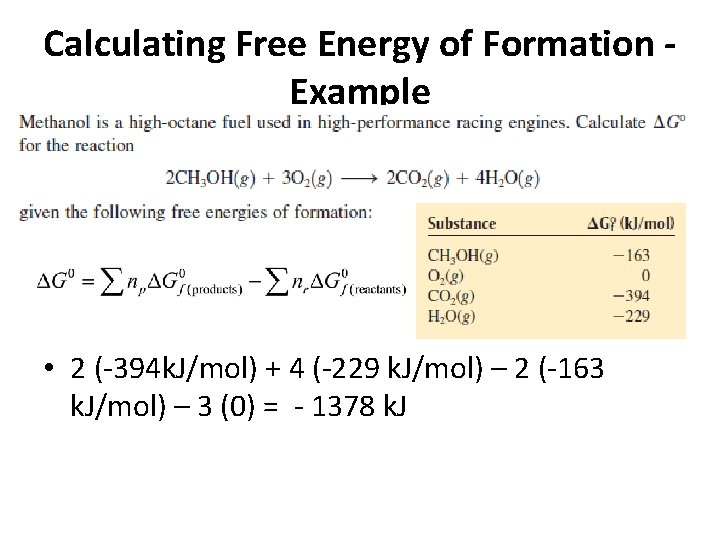
Calculating Free Energy of Formation - Example • 2 (-394 k. J/mol) + 4 (-229 k. J/mol) – 2 (-163 k. J/mol) – 3 (0) = - 1378 k. J
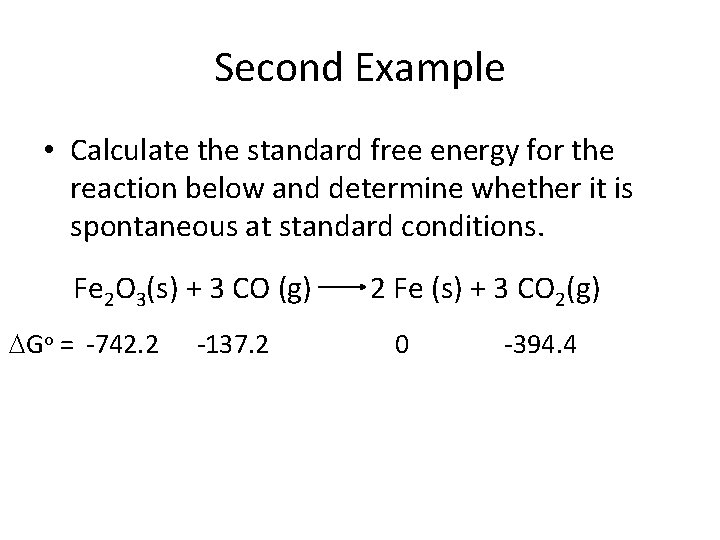
Second Example • Calculate the standard free energy for the reaction below and determine whether it is spontaneous at standard conditions. Fe 2 O 3(s) + 3 CO (g) Go = -742. 2 -137. 2 2 Fe (s) + 3 CO 2(g) 0 -394. 4
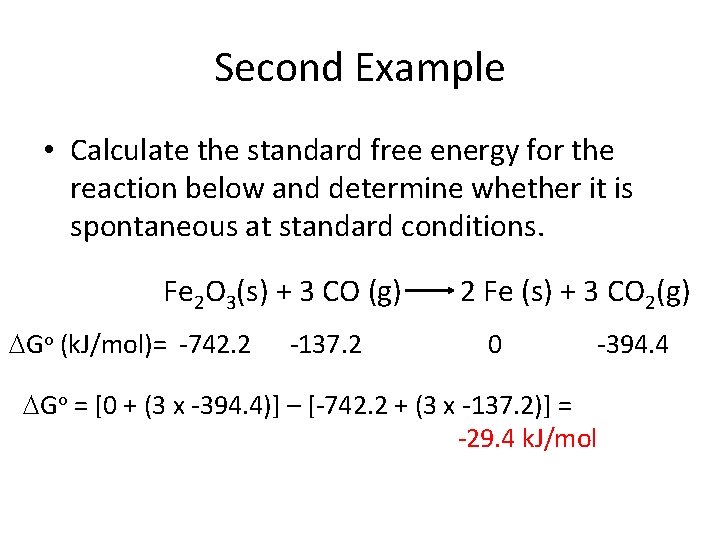
Second Example • Calculate the standard free energy for the reaction below and determine whether it is spontaneous at standard conditions. Fe 2 O 3(s) + 3 CO (g) Go (k. J/mol)= -742. 2 -137. 2 2 Fe (s) + 3 CO 2(g) 0 -394. 4 Go = [0 + (3 x -394. 4)] – [-742. 2 + (3 x -137. 2)] = -29. 4 k. J/mol
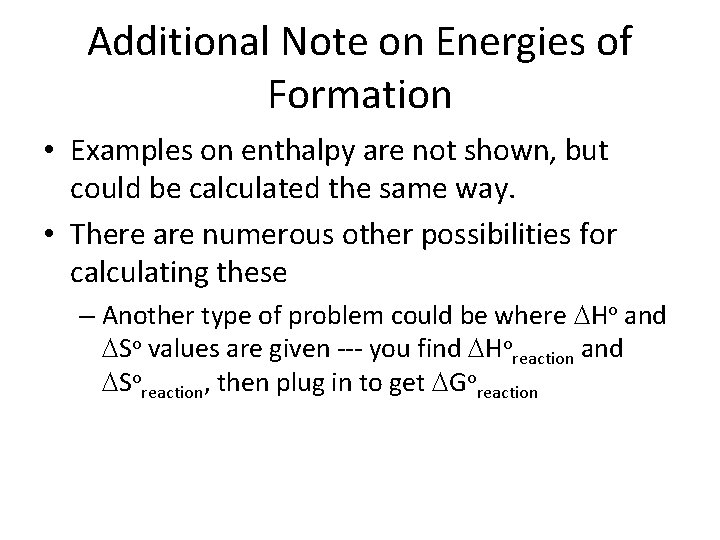
Additional Note on Energies of Formation • Examples on enthalpy are not shown, but could be calculated the same way. • There are numerous other possibilities for calculating these – Another type of problem could be where Ho and So values are given --- you find Horeaction and Soreaction, then plug in to get Goreaction
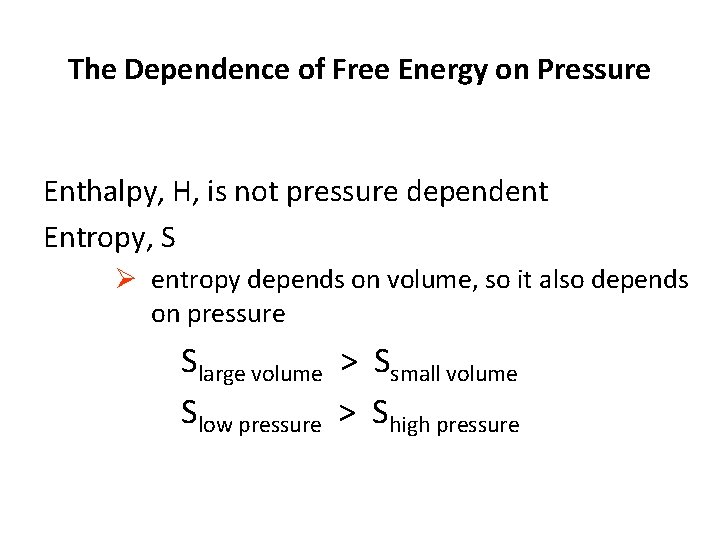
The Dependence of Free Energy on Pressure Enthalpy, H, is not pressure dependent Entropy, S Ø entropy depends on volume, so it also depends on pressure Slarge volume > Ssmall volume Slow pressure > Shigh pressure
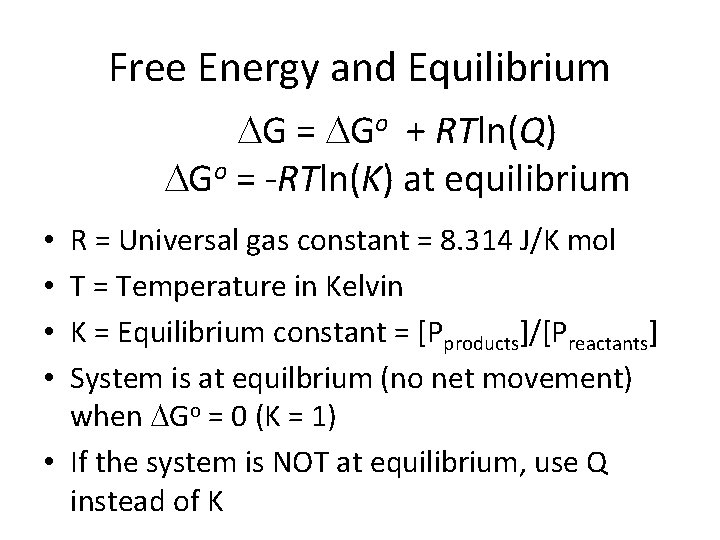
Free Energy and Equilibrium G = Go + RTln(Q) Go = -RTln(K) at equilibrium R = Universal gas constant = 8. 314 J/K mol T = Temperature in Kelvin K = Equilibrium constant = [Pproducts]/[Preactants] System is at equilbrium (no net movement) when Go = 0 (K = 1) • If the system is NOT at equilibrium, use Q instead of K • •
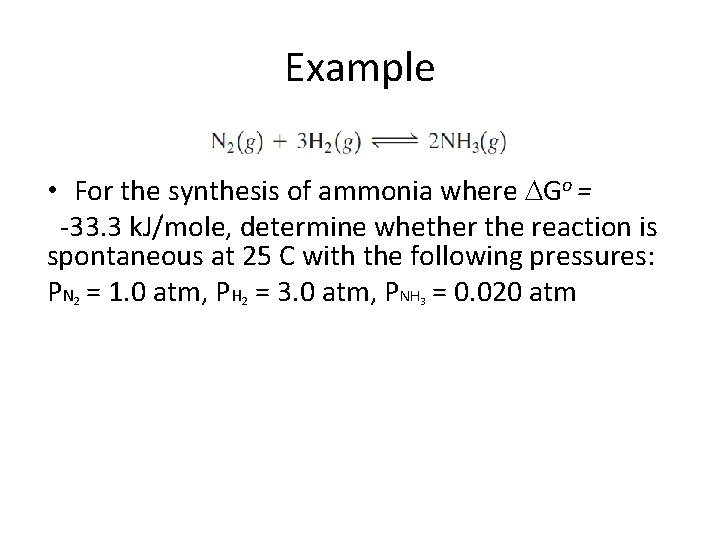
Example • For the synthesis of ammonia where Go = -33. 3 k. J/mole, determine whether the reaction is spontaneous at 25 C with the following pressures: PN = 1. 0 atm, PH = 3. 0 atm, PNH = 0. 020 atm 2 2 3
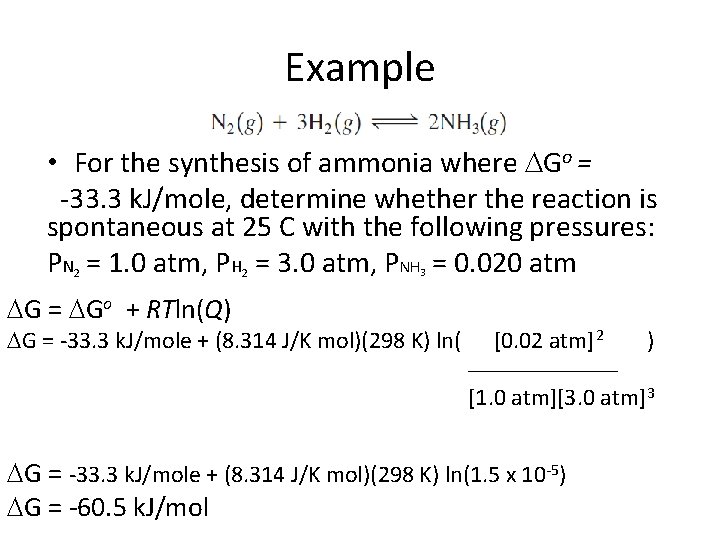
Example • For the synthesis of ammonia where Go = -33. 3 k. J/mole, determine whether the reaction is spontaneous at 25 C with the following pressures: PN = 1. 0 atm, PH = 3. 0 atm, PNH = 0. 020 atm 2 G = Go + RTln(Q) 2 3 G = -33. 3 k. J/mole + (8. 314 J/K mol)(298 K) ln( [0. 02 atm]2 ) __________ [1. 0 atm][3. 0 atm]3 G = -33. 3 k. J/mole + (8. 314 J/K mol)(298 K) ln(1. 5 x 10 -5) G = -60. 5 k. J/mol
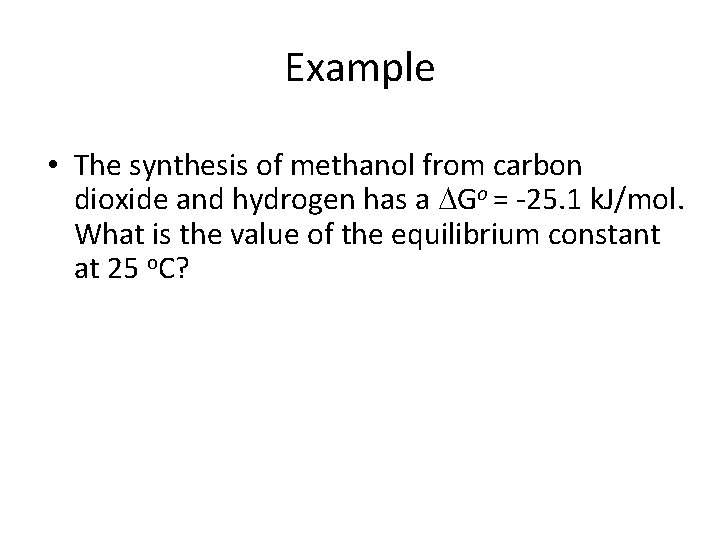
Example • The synthesis of methanol from carbon dioxide and hydrogen has a Go = -25. 1 k. J/mol. What is the value of the equilibrium constant at 25 o. C?
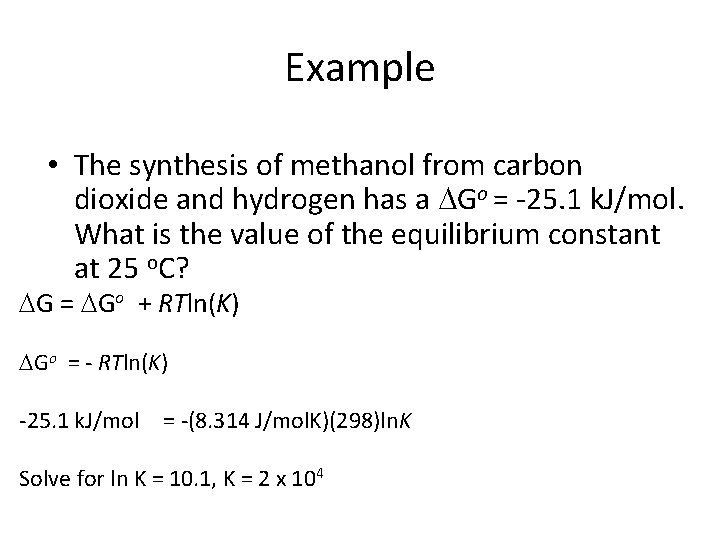
Example • The synthesis of methanol from carbon dioxide and hydrogen has a Go = -25. 1 k. J/mol. What is the value of the equilibrium constant at 25 o. C? G = Go + RTln(K) Go = - RTln(K) -25. 1 k. J/mol = -(8. 314 J/mol. K)(298)ln. K Solve for ln K = 10. 1, K = 2 x 104
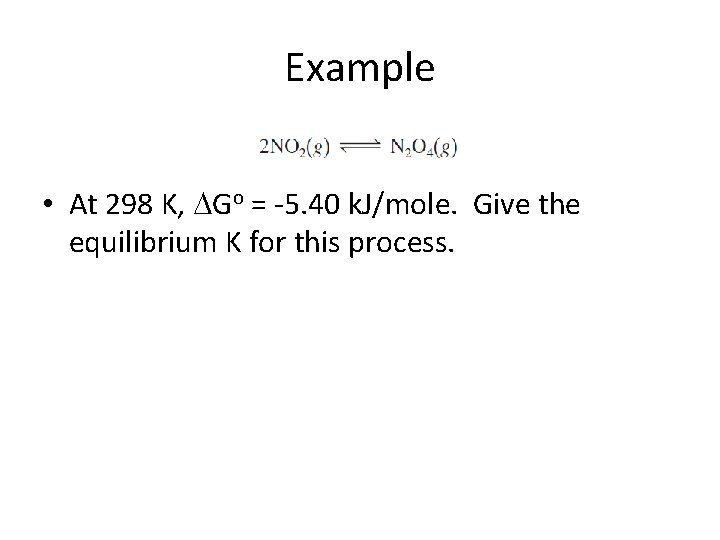
Example • At 298 K, Go = -5. 40 k. J/mole. Give the equilibrium K for this process.
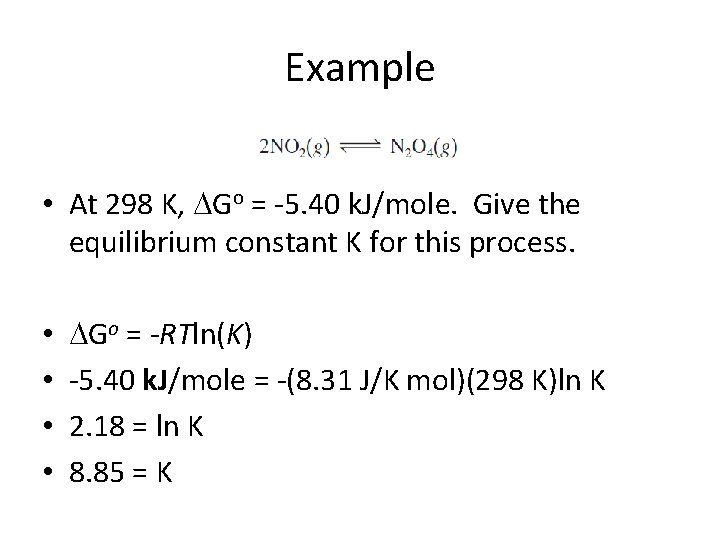
Example • At 298 K, Go = -5. 40 k. J/mole. Give the equilibrium constant K for this process. • • Go = -RTln(K) -5. 40 k. J/mole = -(8. 31 J/K mol)(298 K)ln K 2. 18 = ln K 8. 85 = K
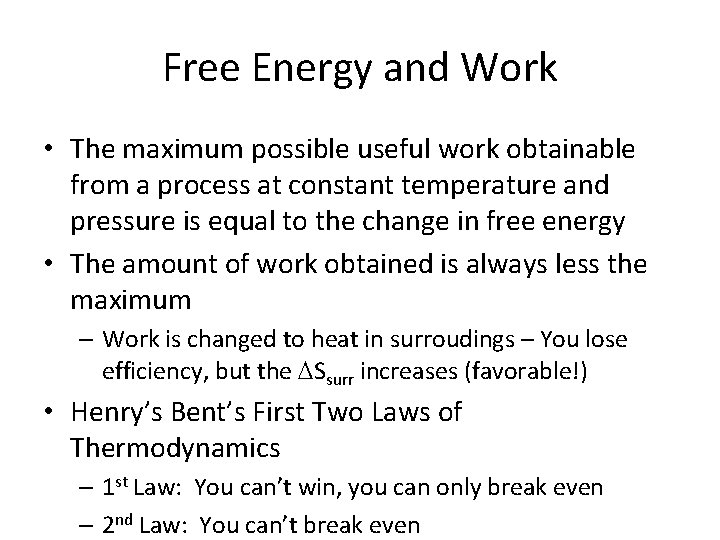
Free Energy and Work • The maximum possible useful work obtainable from a process at constant temperature and pressure is equal to the change in free energy • The amount of work obtained is always less the maximum – Work is changed to heat in surroudings – You lose efficiency, but the Ssurr increases (favorable!) • Henry’s Bent’s First Two Laws of Thermodynamics – 1 st Law: You can’t win, you can only break even – 2 nd Law: You can’t break even
Ap chem spontaneity entropy and free energy
Chemistry microstates
Gibbs free energy non standard conditions
Gibbs free energy non standard conditions
Relationship between entropy and free energy
Enthalpy entropy free energy
Helmholtz free energy
Positive delta g spontaneous
Enthalpy entropy free energy
How to find free energy
Rt ln k
Thermodynamics ppt
Predicting spontaneity
Concentration cell
Predicting spontaneity
Predicting spontaneity
Redox table
Energy energy transfer and general energy analysis
Energy energy transfer and general energy analysis
Free body and soul free
Free hearts free foreheads
Entropy and heat transfer
What is enthalpy and entropy
Minimum enthalpy maximum entropy
Entropy system and surroundings
Entropy order parameters and complexity
Chapter 7 energy conservation of energy
Allocation map
Free free absorption
Phospho anhydride
Entropy equation temperature
The increase of entropy principle
Energi bebas gibbs
Spontaneous irreversible process
Isentropic process
Entropy is scalar or vector
δhsys
Entropy thermodynamics
Entropy change formula
Entropy in bits
Huffman tree visualization
Entropy rate balance
Classical entropy
Entropy change at constant volume formula
Physical science chapter 6 review answers
Standard entropy change formula
Refrigerator entropy
Units of entropu
Entropy simple definition
G=h-ts
Entropy = q/t