What Well Use General Chemistry Physics Physical Chemistry
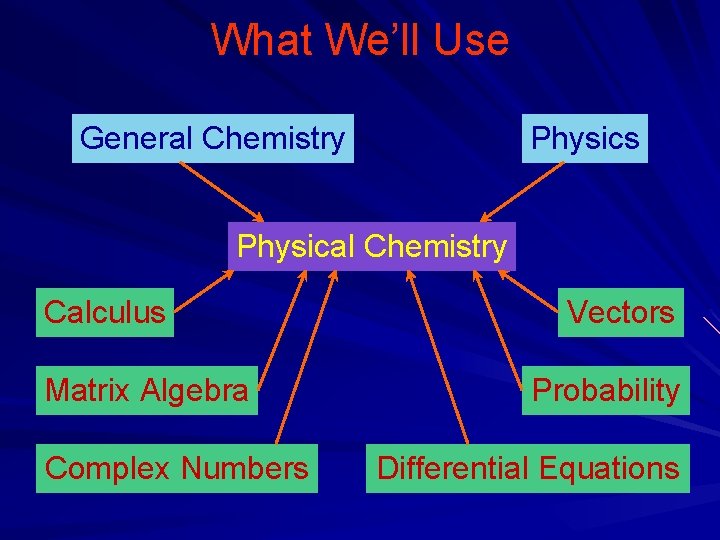
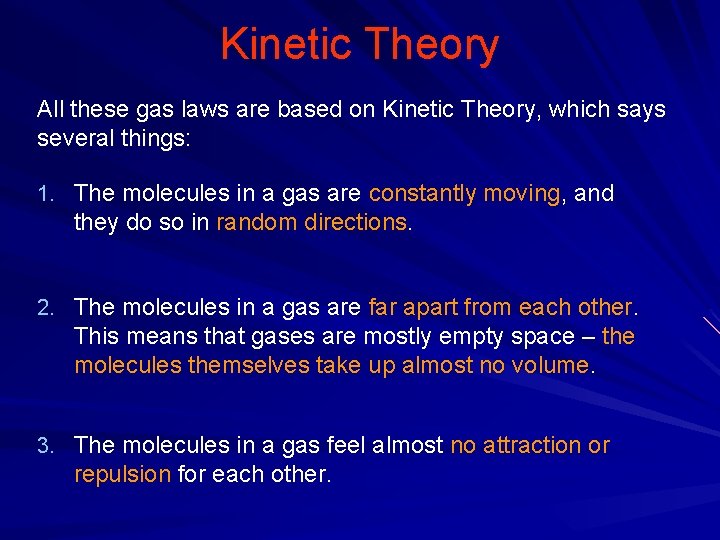
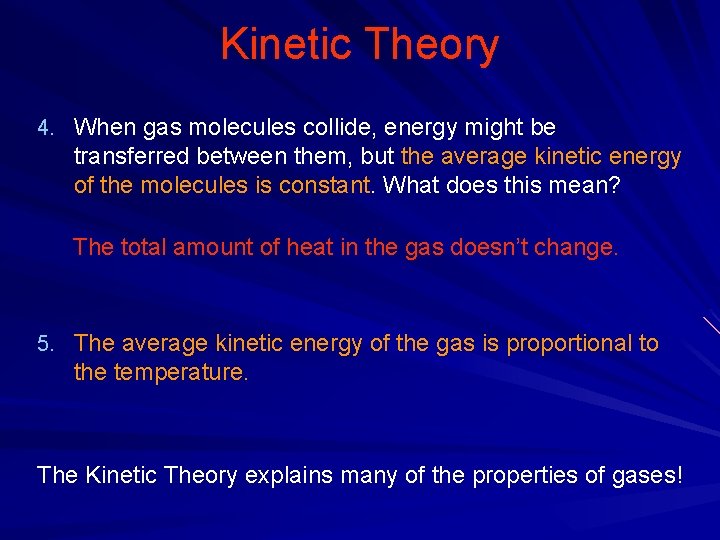
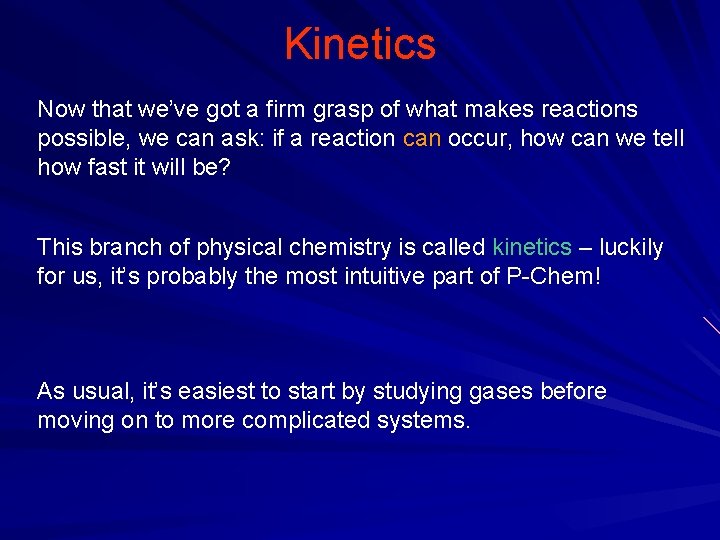
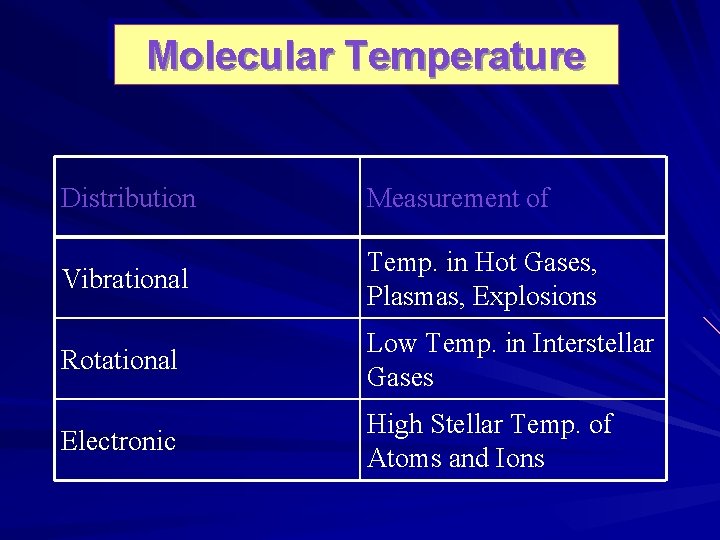
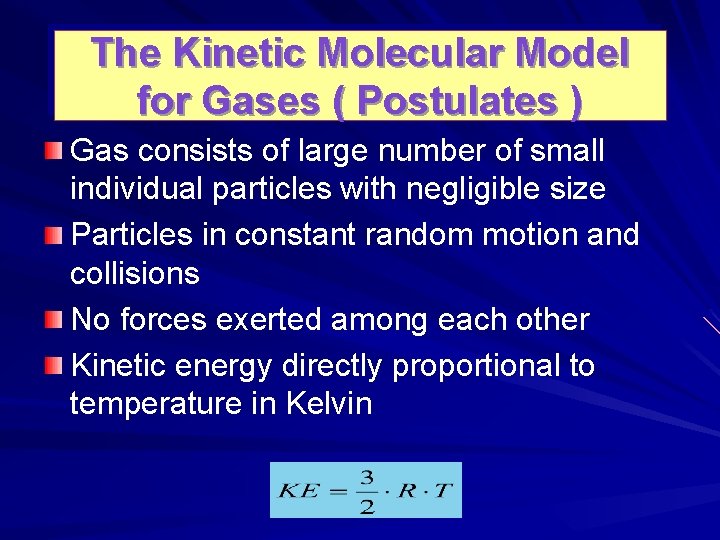
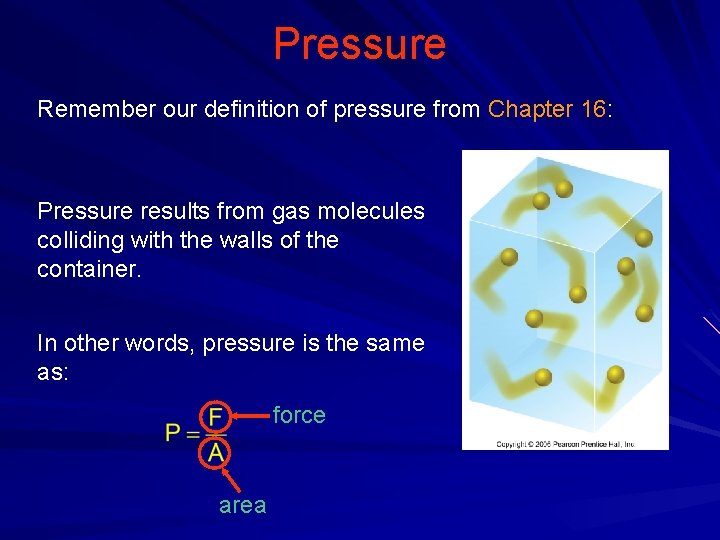
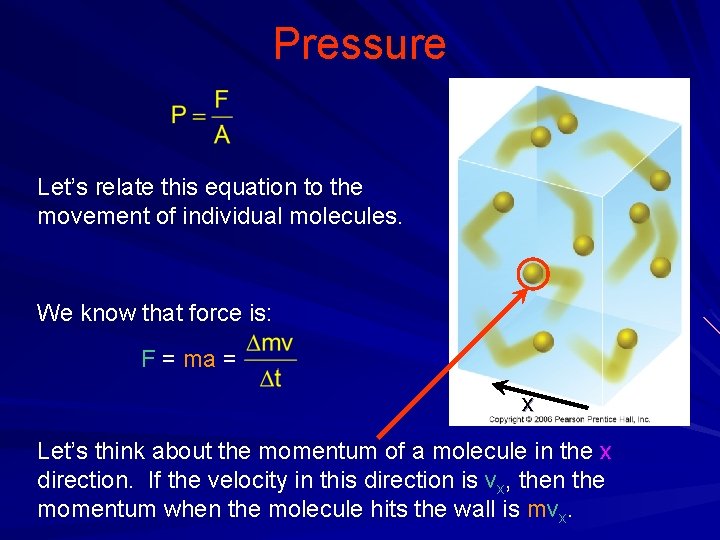
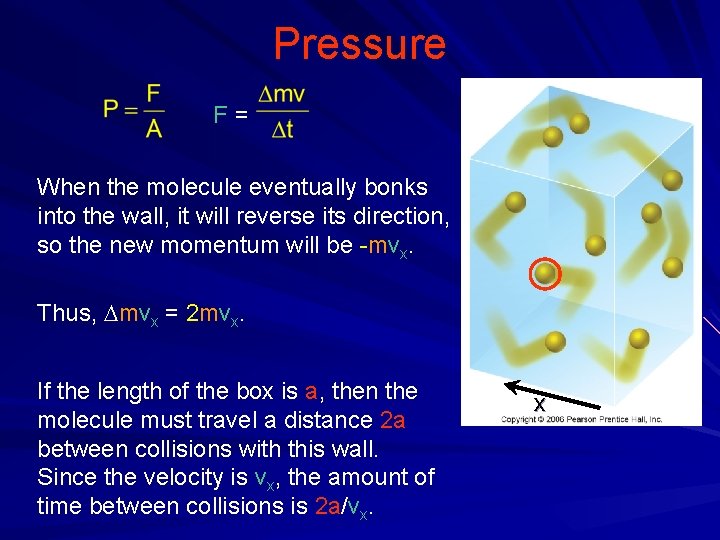
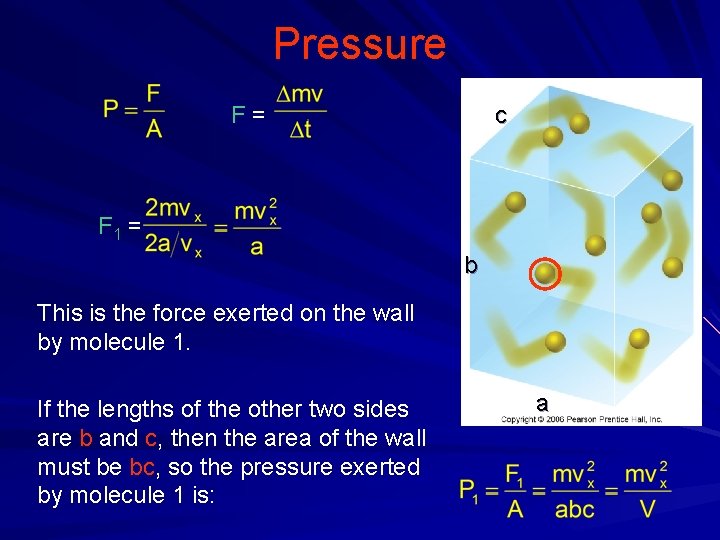
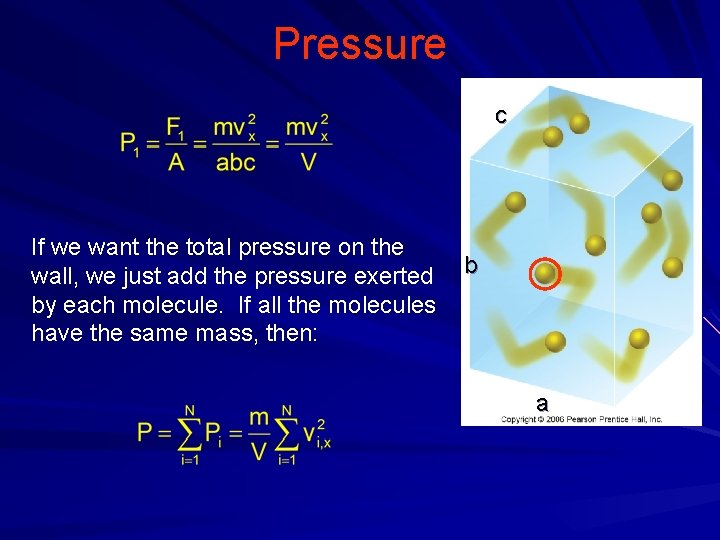
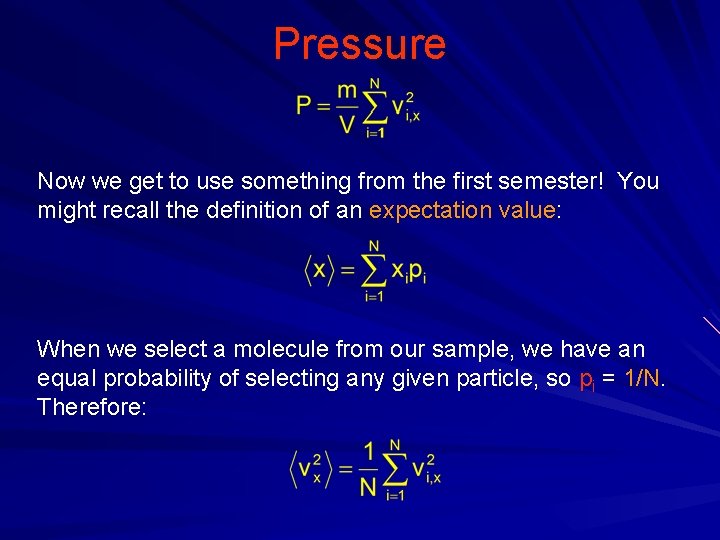
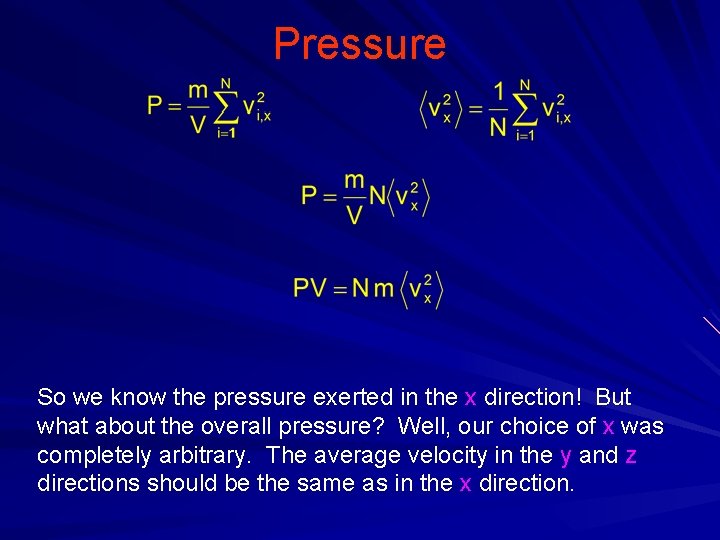
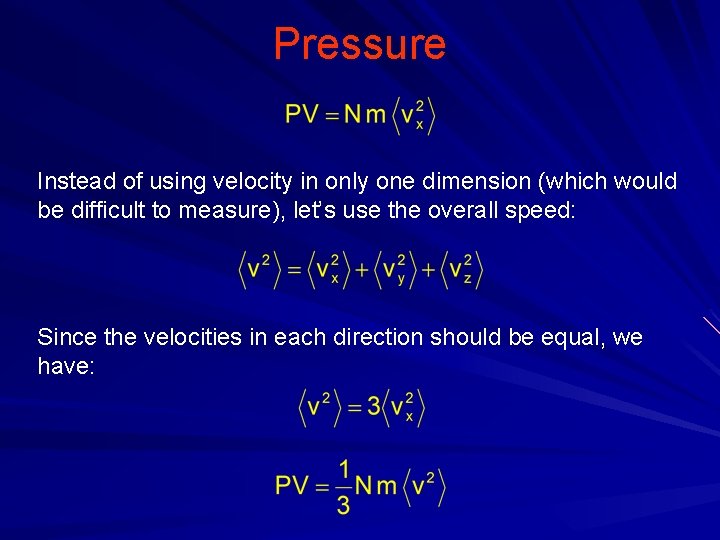
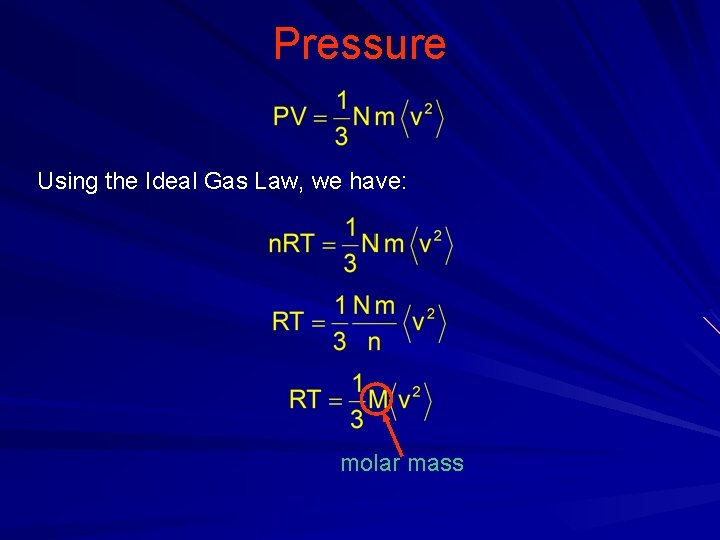
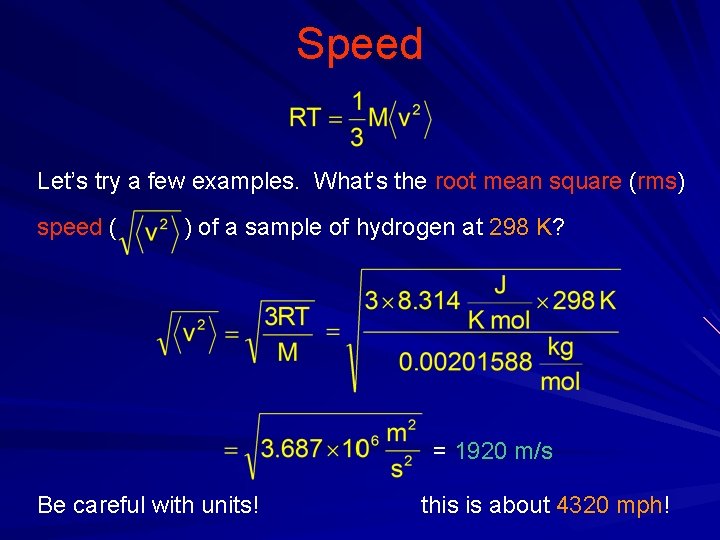
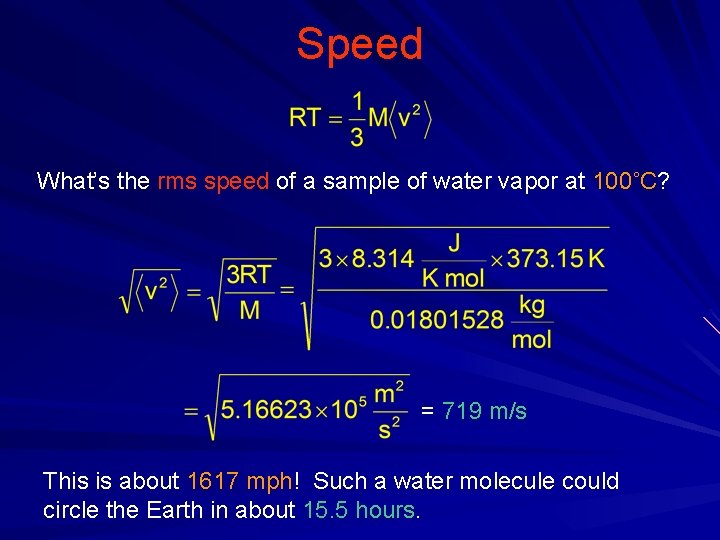
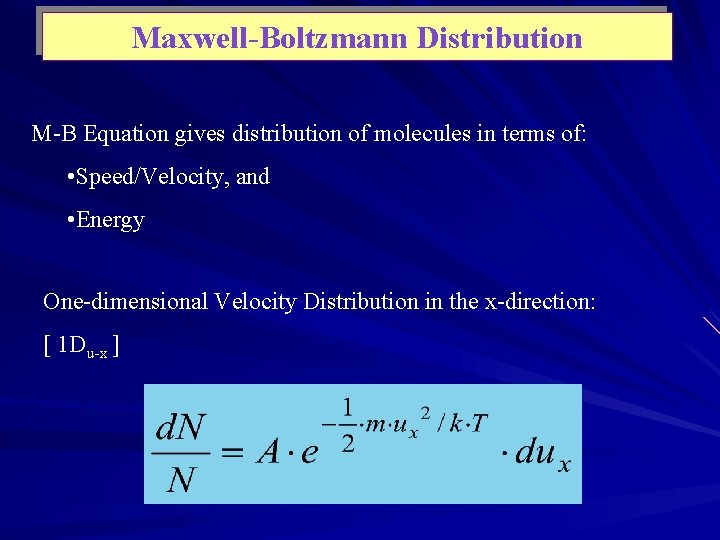
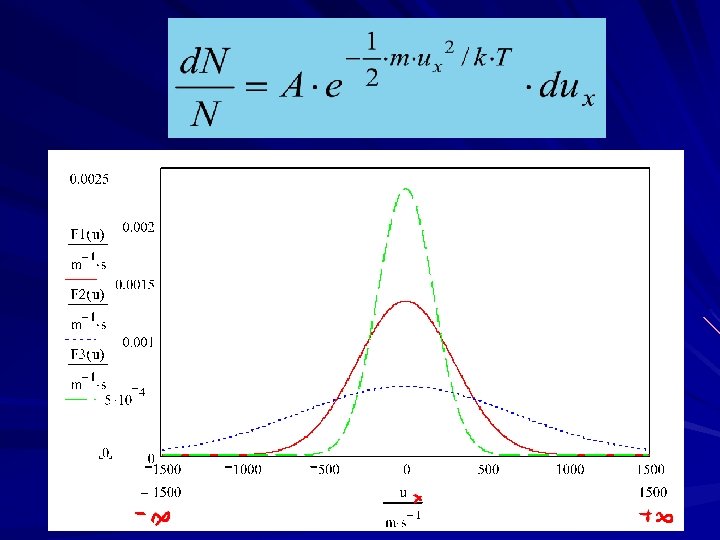
![3 D Maxwell-Boltzmann Distribution 3 D Velocity Distribution: Cartesian Coordinates: [ 3 Du ] 3 D Maxwell-Boltzmann Distribution 3 D Velocity Distribution: Cartesian Coordinates: [ 3 Du ]](https://slidetodoc.com/presentation_image_h2/8561b2bf76979d16c978df483d4fe6d2/image-20.jpg)
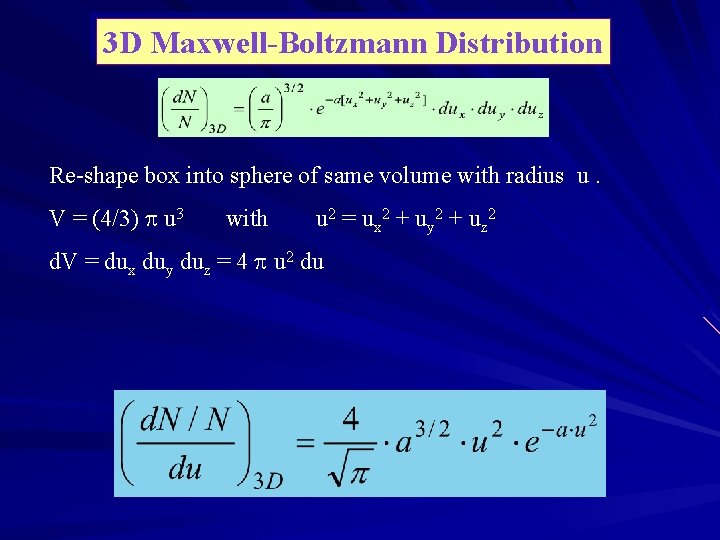
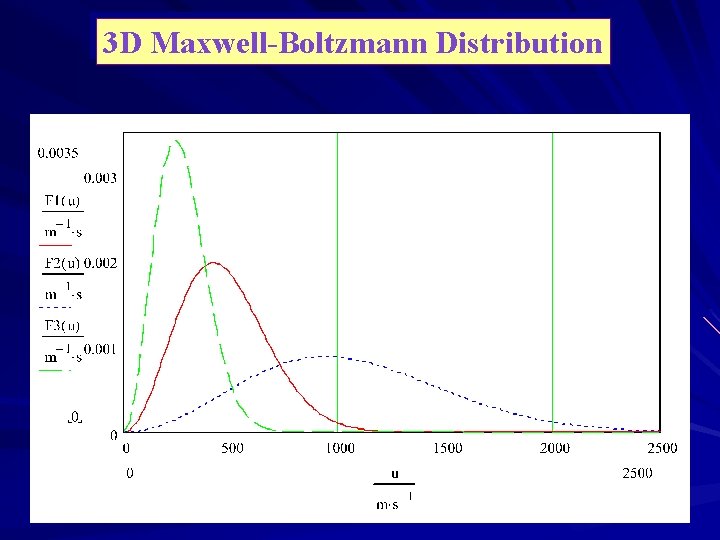
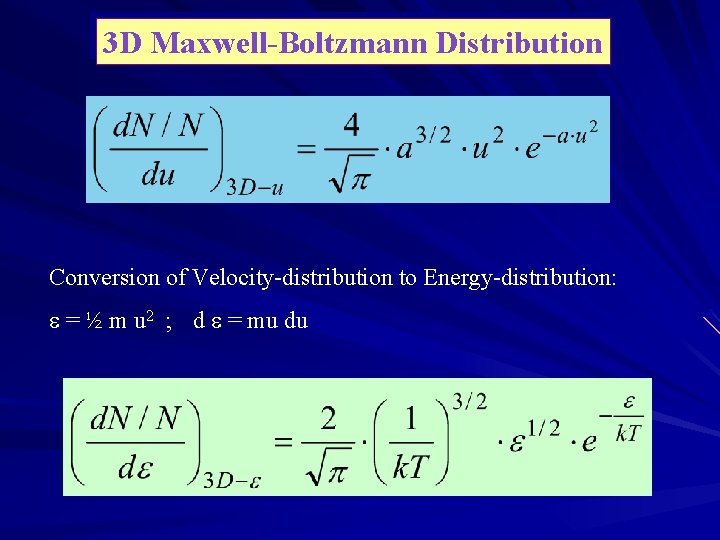
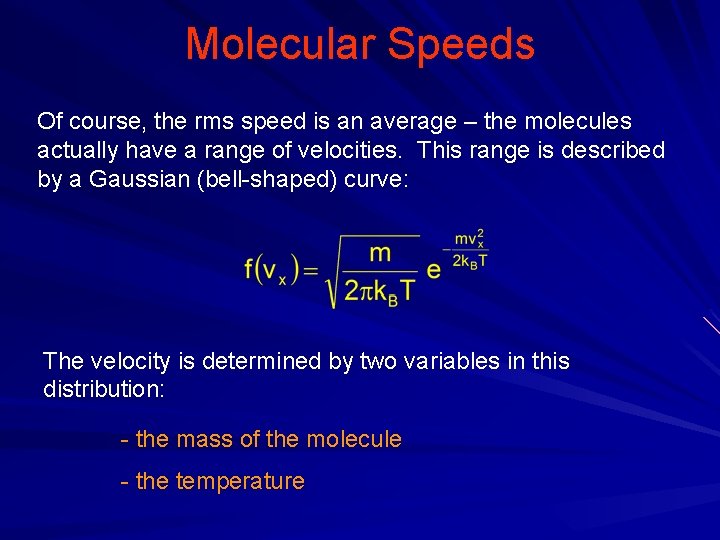
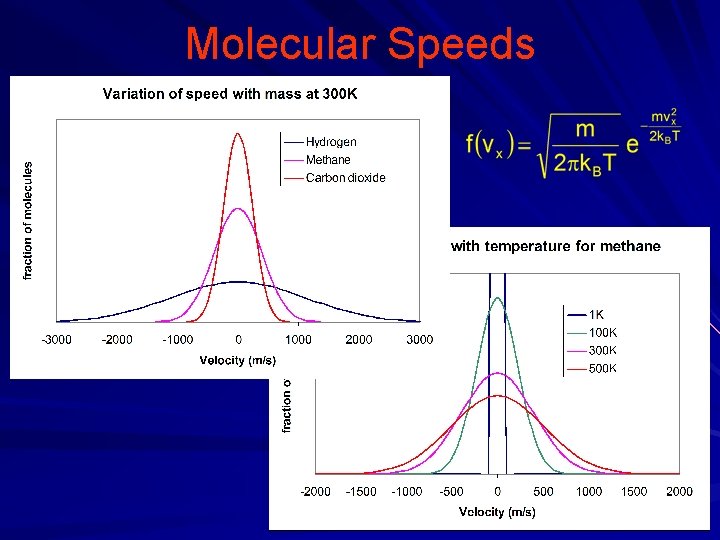
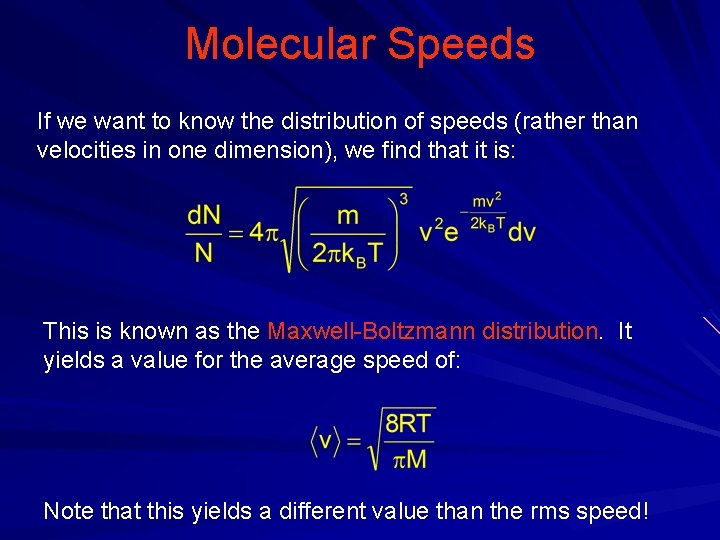
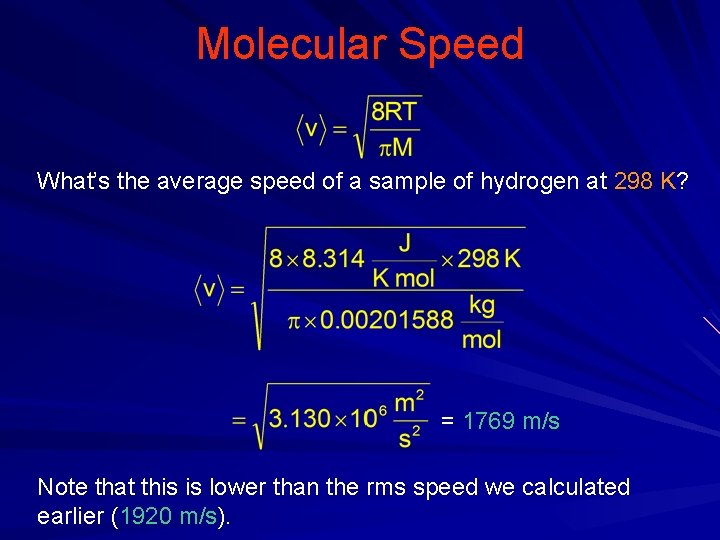
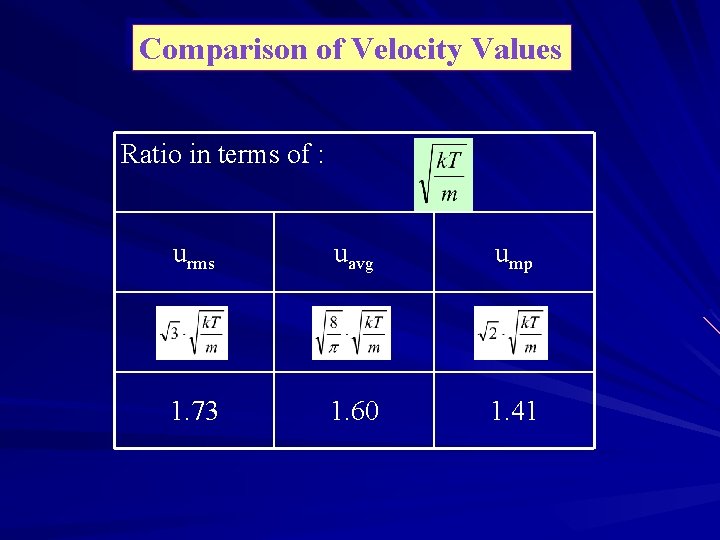
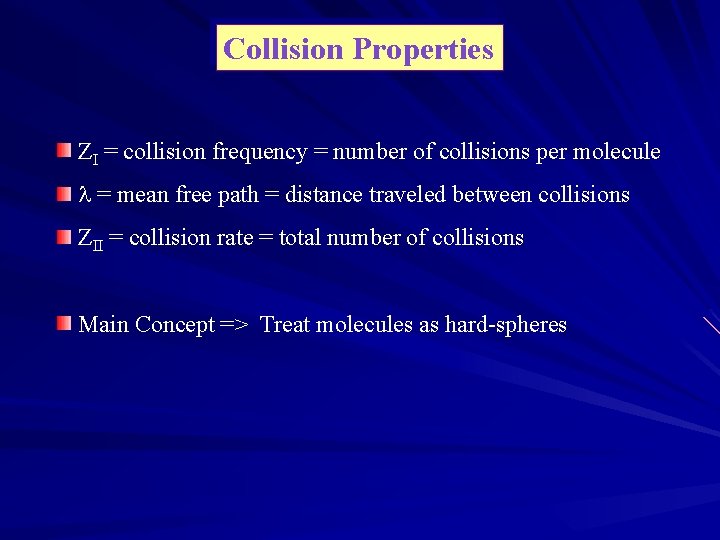
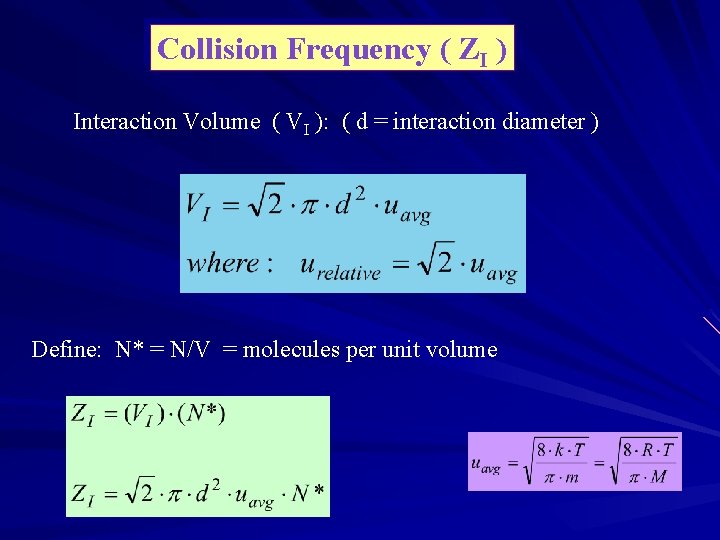
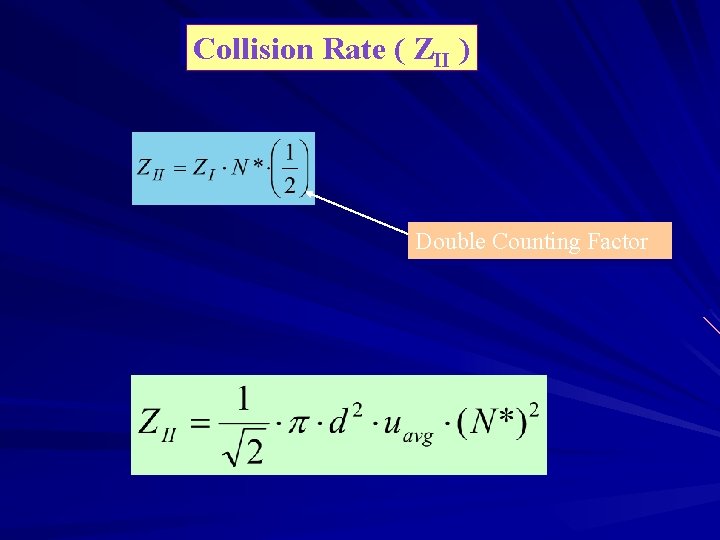
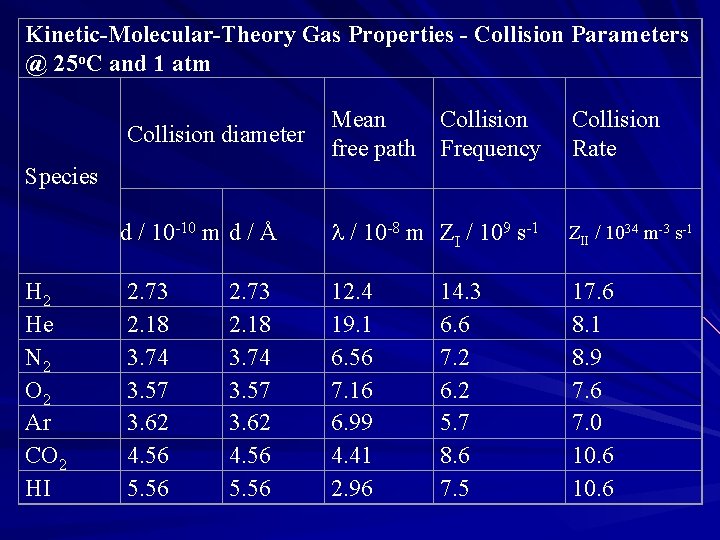
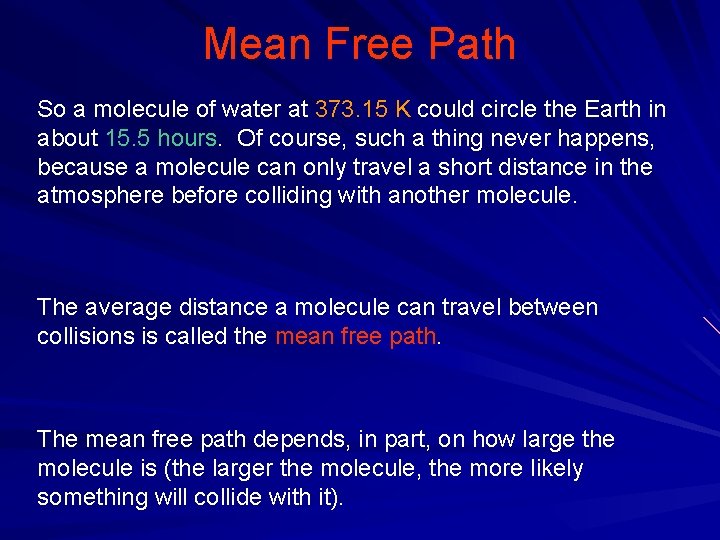
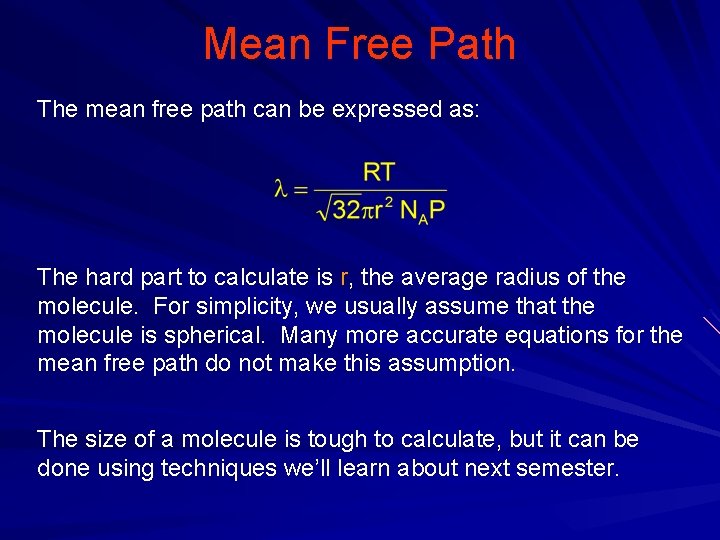
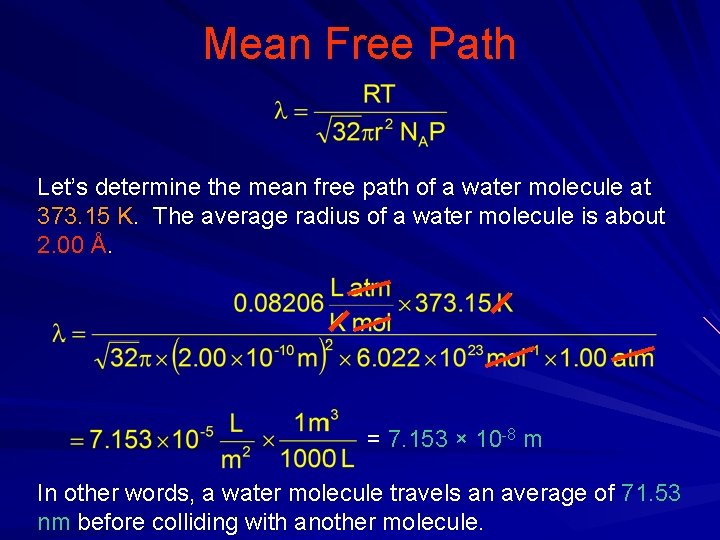
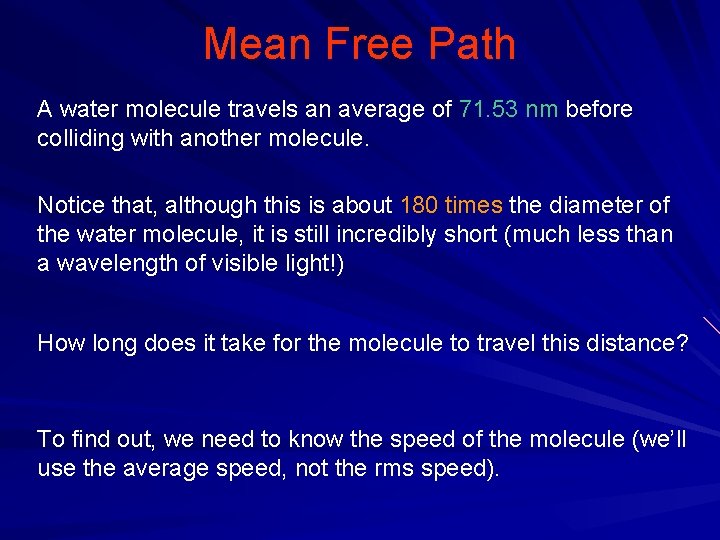
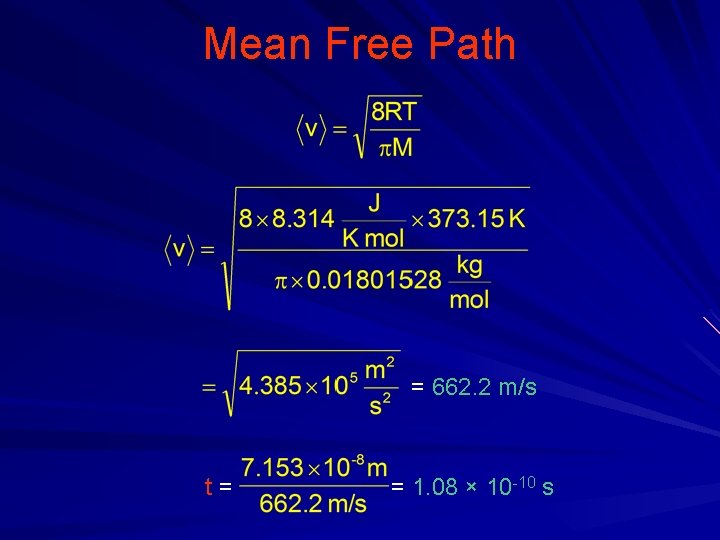
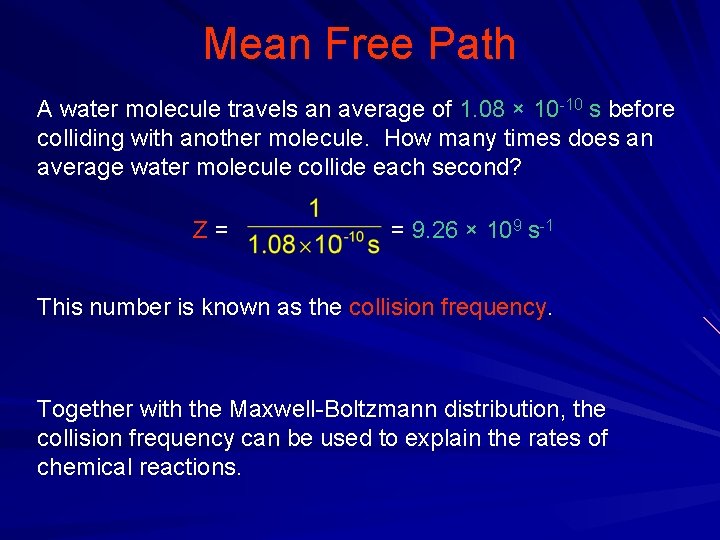
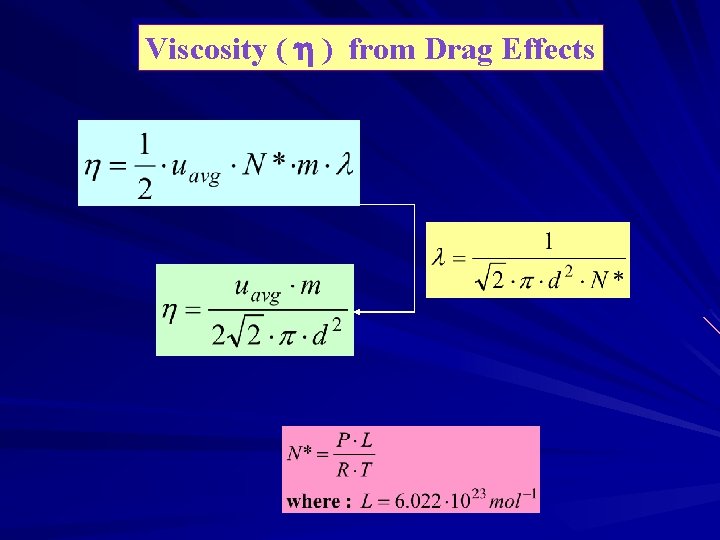
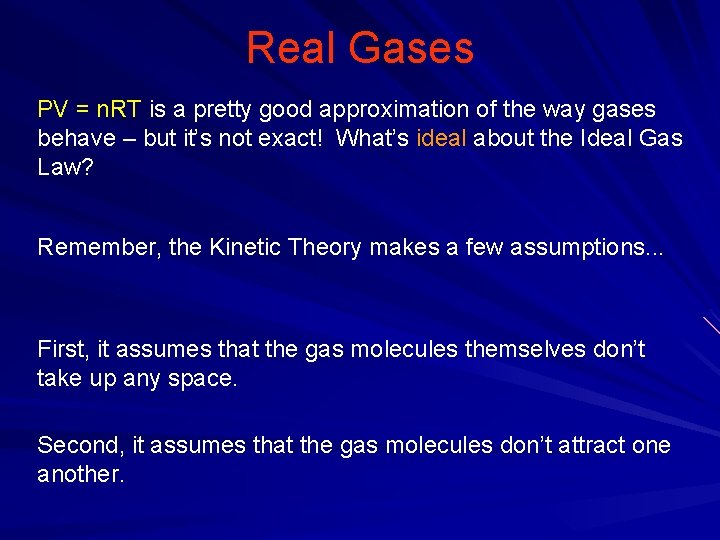
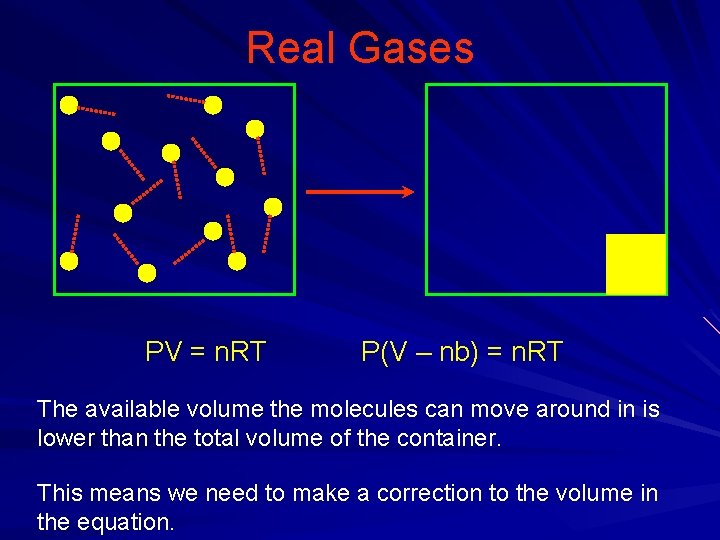
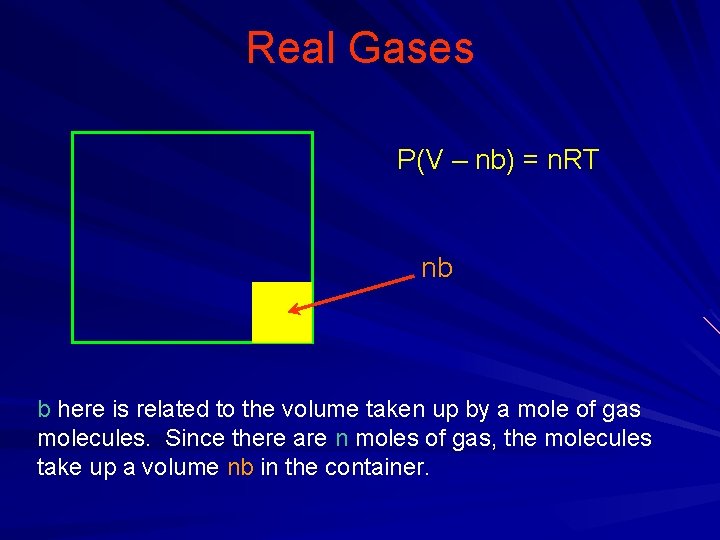
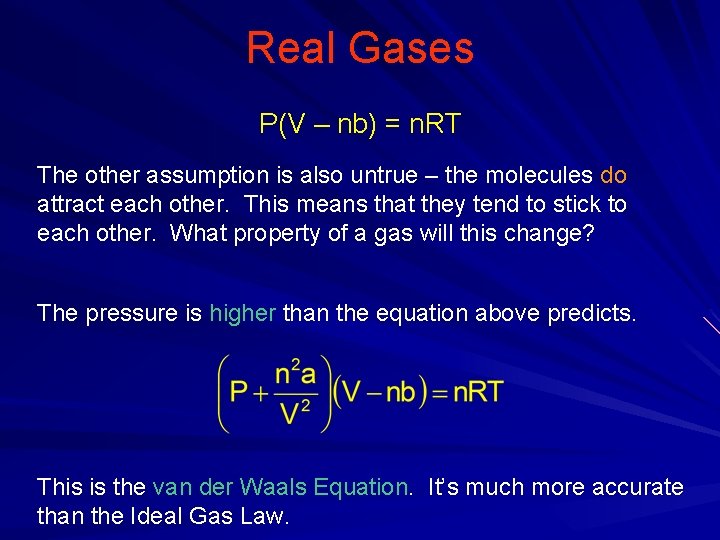
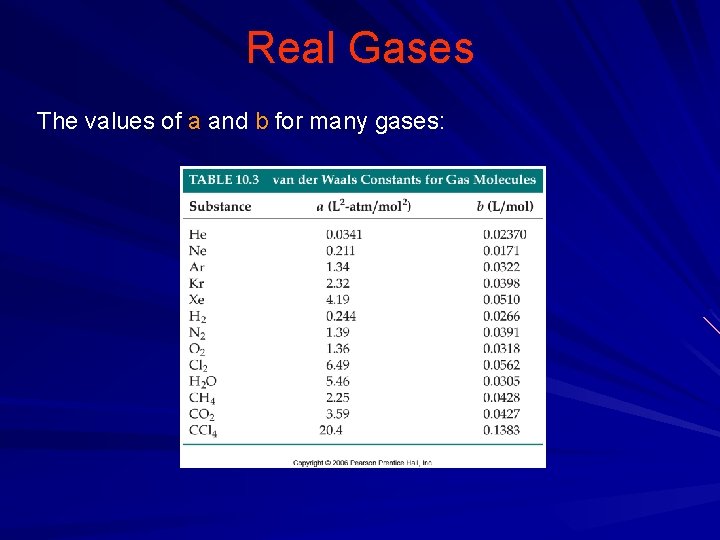
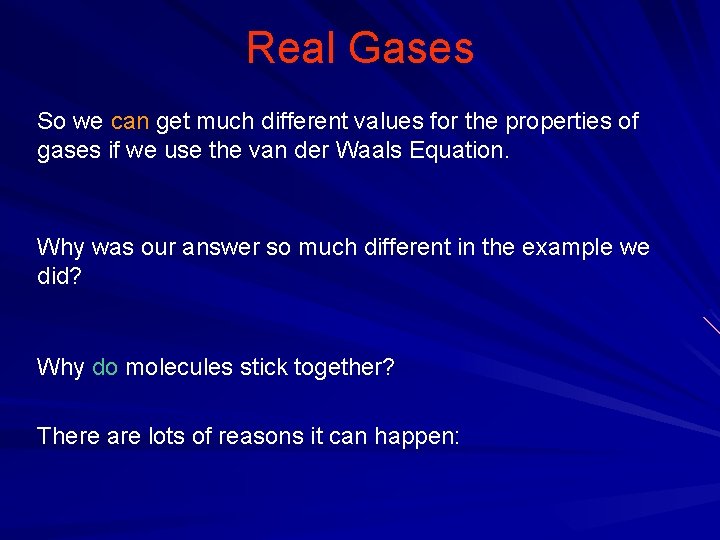
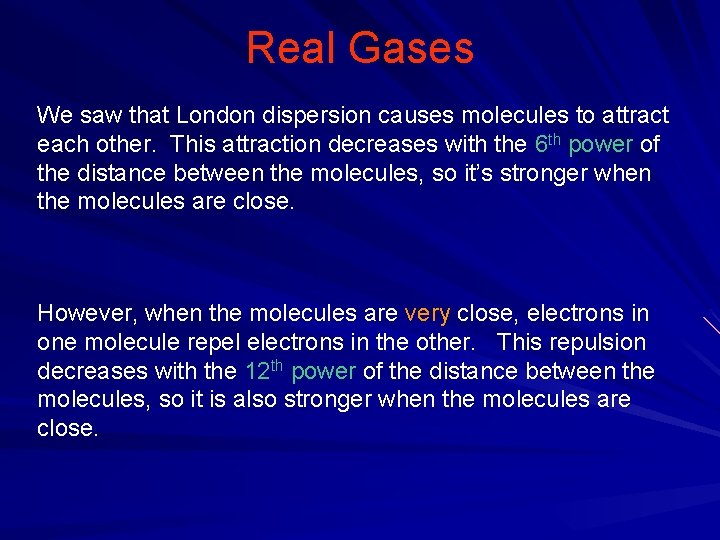
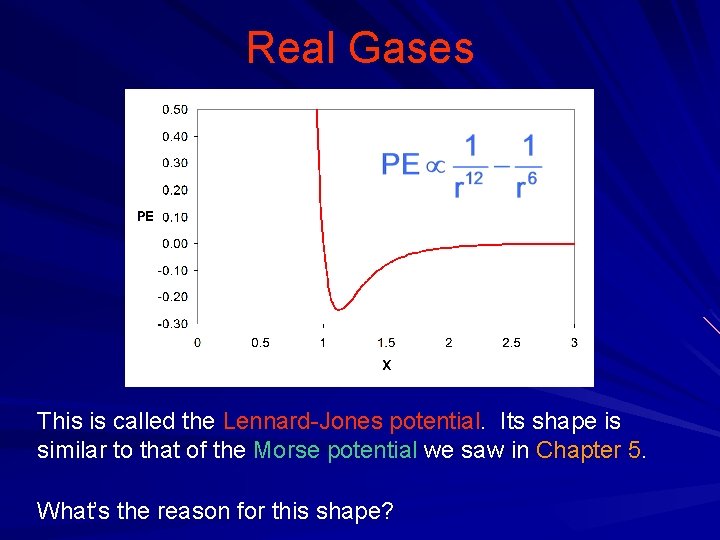
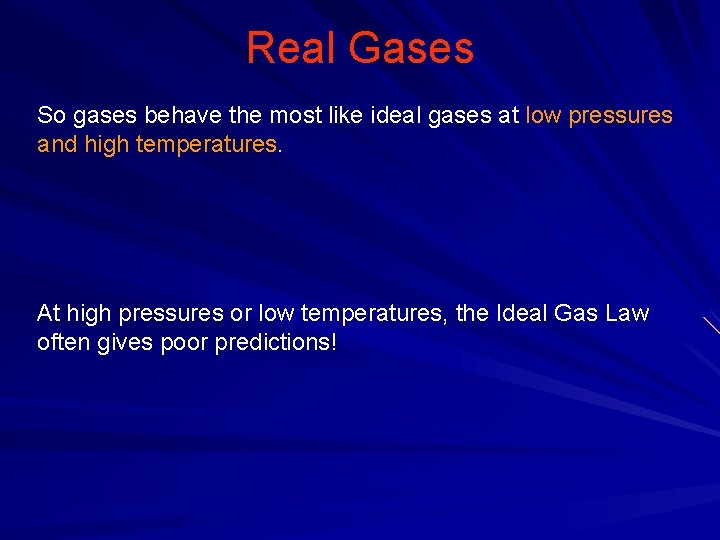
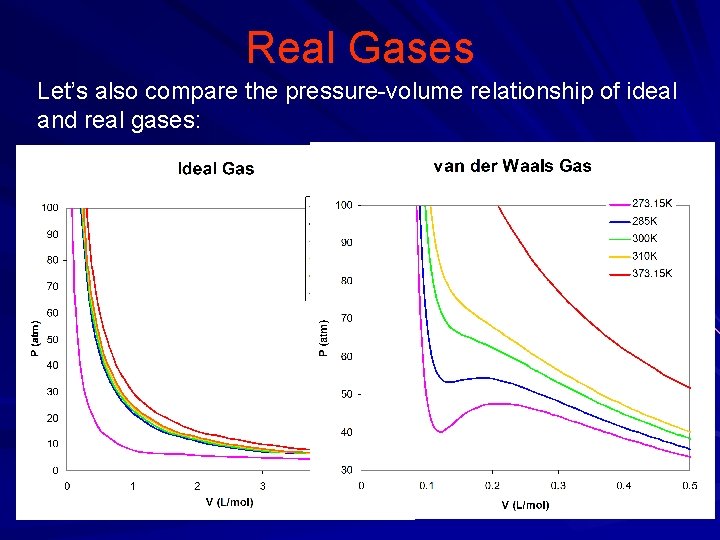
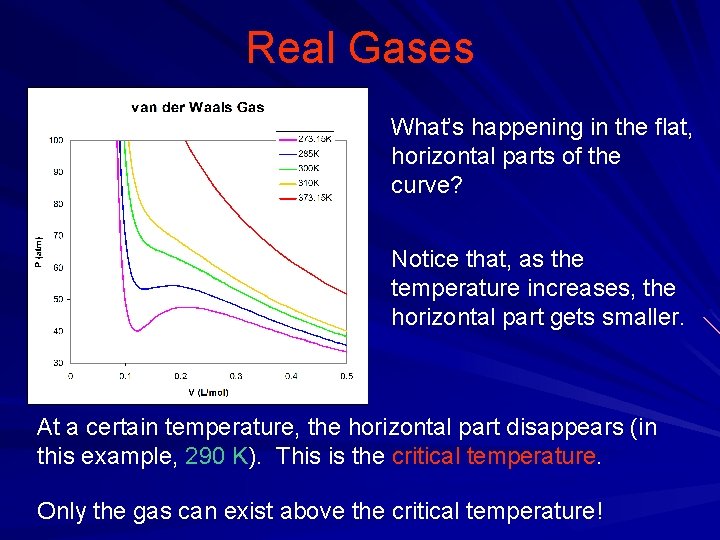
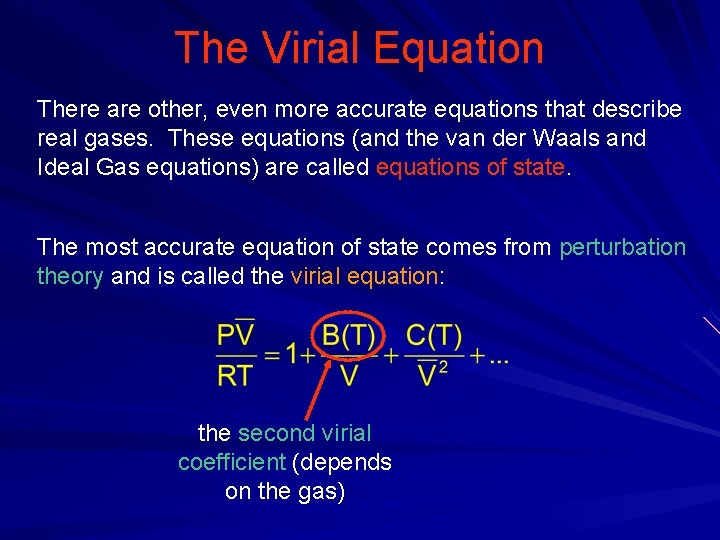
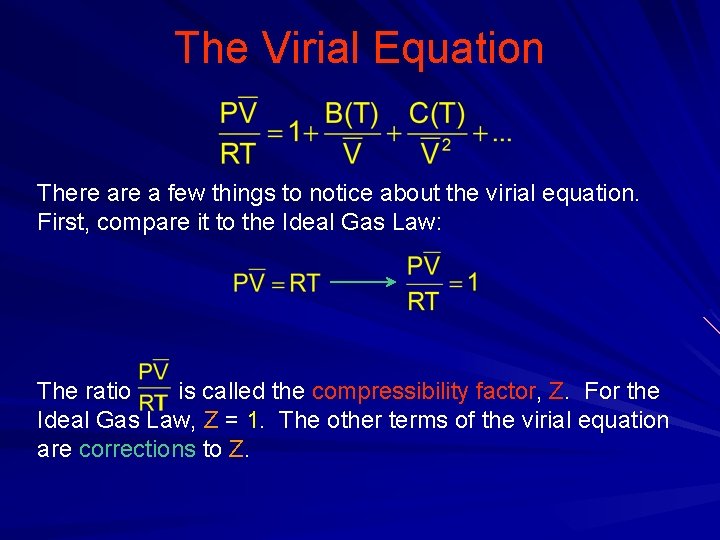
- Slides: 52
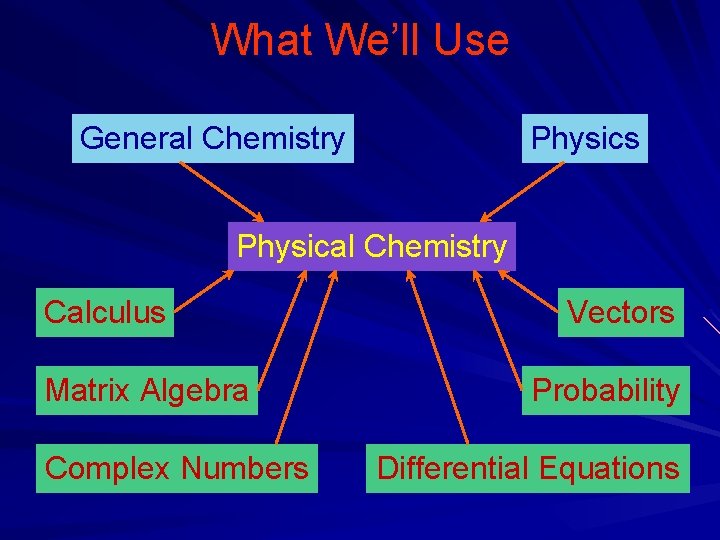
What We’ll Use General Chemistry Physics Physical Chemistry Calculus Matrix Algebra Complex Numbers Vectors Probability Differential Equations
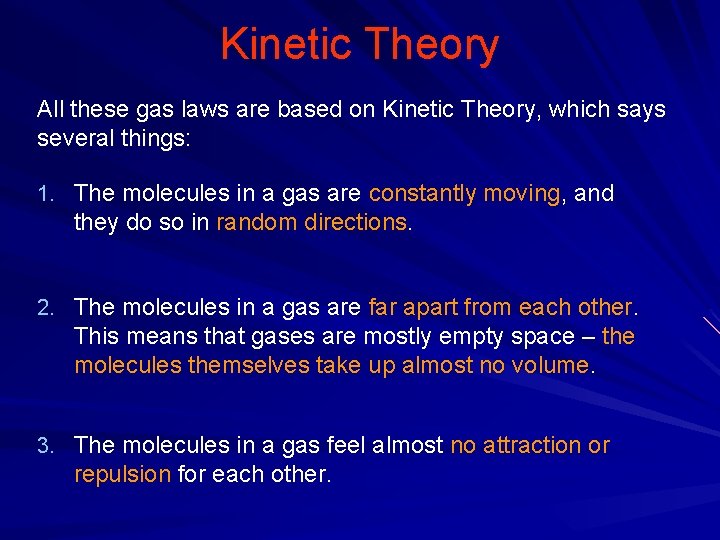
Kinetic Theory All these gas laws are based on Kinetic Theory, which says several things: 1. The molecules in a gas are constantly moving, and they do so in random directions. 2. The molecules in a gas are far apart from each other. This means that gases are mostly empty space – the molecules themselves take up almost no volume. 3. The molecules in a gas feel almost no attraction or repulsion for each other.
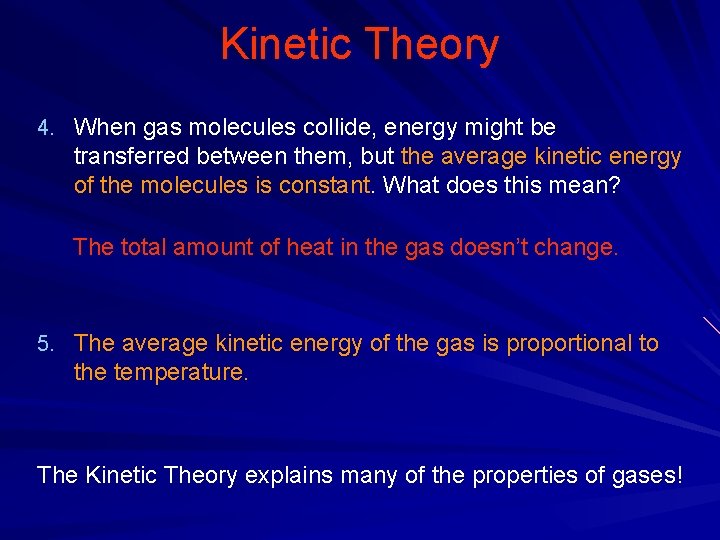
Kinetic Theory 4. When gas molecules collide, energy might be transferred between them, but the average kinetic energy of the molecules is constant. What does this mean? The total amount of heat in the gas doesn’t change. 5. The average kinetic energy of the gas is proportional to the temperature. The Kinetic Theory explains many of the properties of gases!
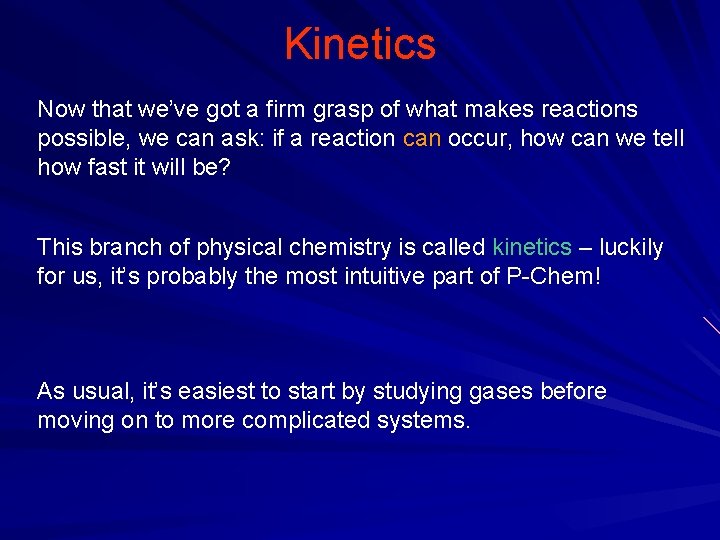
Kinetics Now that we’ve got a firm grasp of what makes reactions possible, we can ask: if a reaction can occur, how can we tell how fast it will be? This branch of physical chemistry is called kinetics – luckily for us, it’s probably the most intuitive part of P-Chem! As usual, it’s easiest to start by studying gases before moving on to more complicated systems.
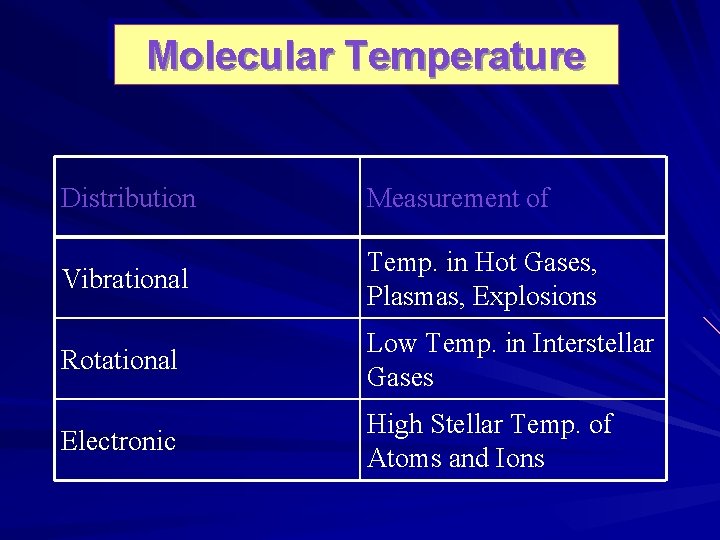
Molecular Temperature Distribution Measurement of Vibrational Temp. in Hot Gases, Plasmas, Explosions Rotational Low Temp. in Interstellar Gases Electronic High Stellar Temp. of Atoms and Ions
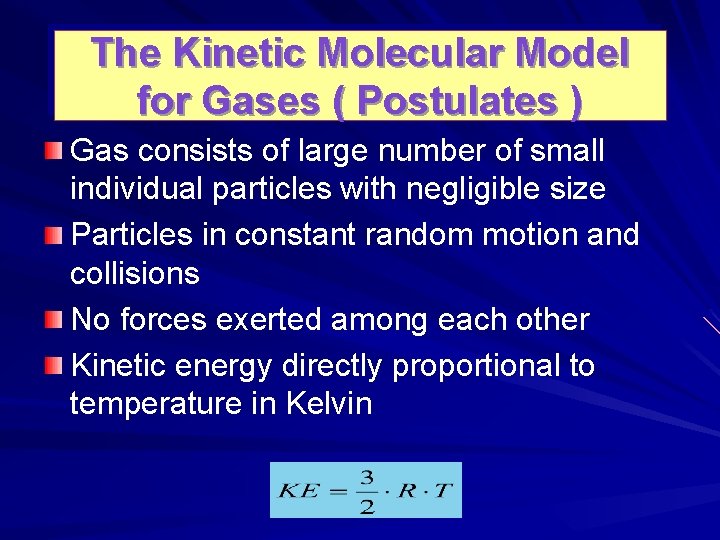
The Kinetic Molecular Model for Gases ( Postulates ) Gas consists of large number of small individual particles with negligible size Particles in constant random motion and collisions No forces exerted among each other Kinetic energy directly proportional to temperature in Kelvin
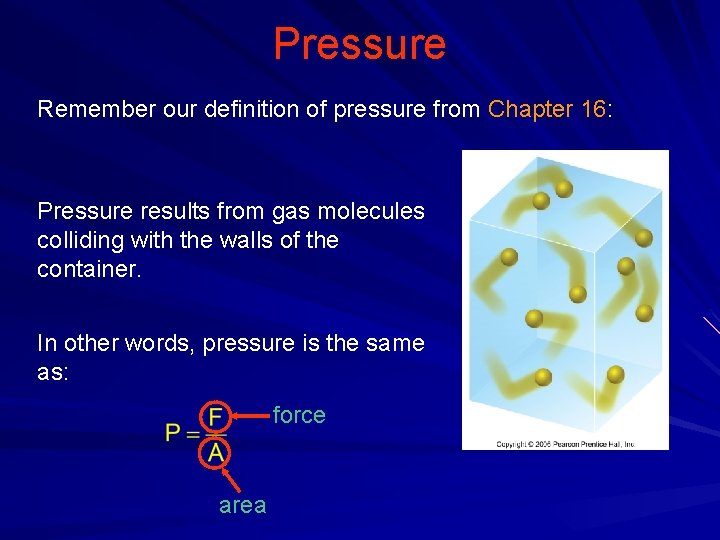
Pressure Remember our definition of pressure from Chapter 16: Pressure results from gas molecules colliding with the walls of the container. In other words, pressure is the same as: force area
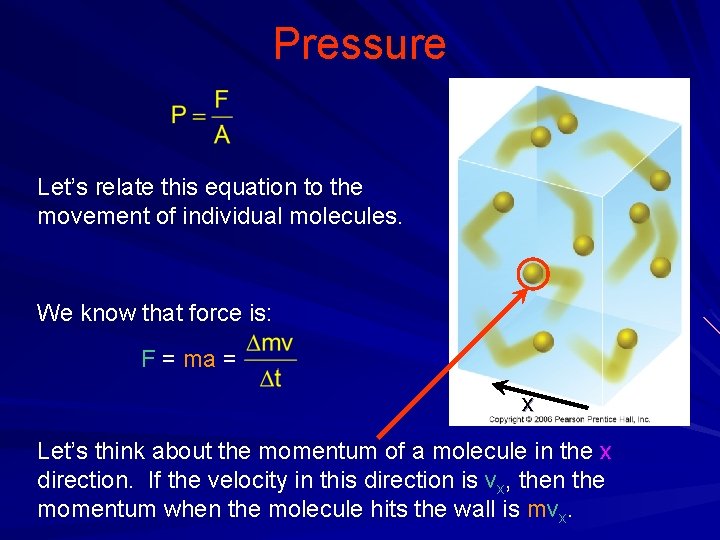
Pressure Let’s relate this equation to the movement of individual molecules. We know that force is: F = ma = x Let’s think about the momentum of a molecule in the x direction. If the velocity in this direction is vx, then the momentum when the molecule hits the wall is mvx.
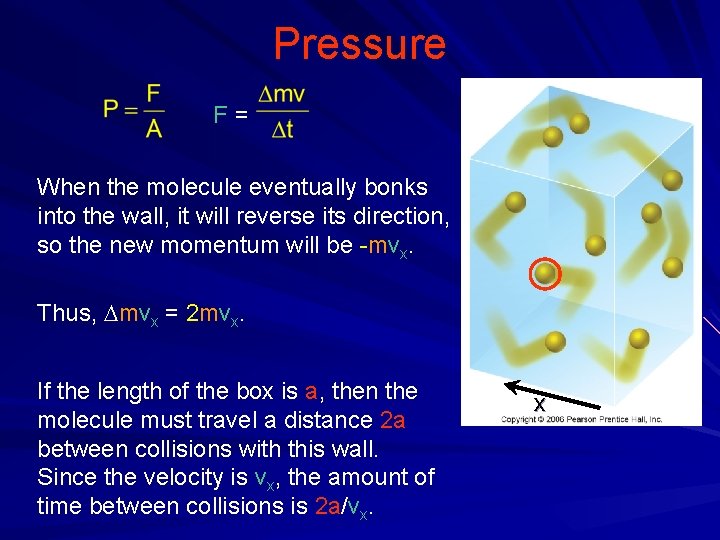
Pressure F= When the molecule eventually bonks into the wall, it will reverse its direction, so the new momentum will be -mvx. Thus, Dmvx = 2 mvx. If the length of the box is a, then the molecule must travel a distance 2 a between collisions with this wall. Since the velocity is vx, the amount of time between collisions is 2 a/vx. x
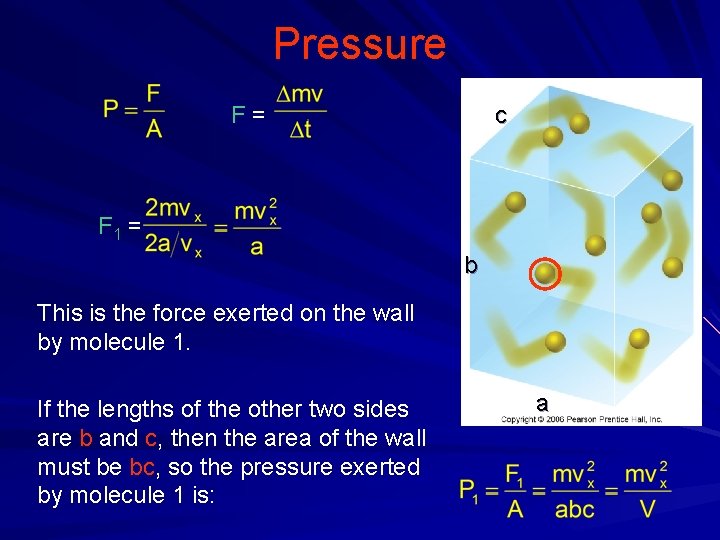
Pressure F= c F 1 = b This is the force exerted on the wall by molecule 1. If the lengths of the other two sides are b and c, then the area of the wall must be bc, so the pressure exerted by molecule 1 is: a
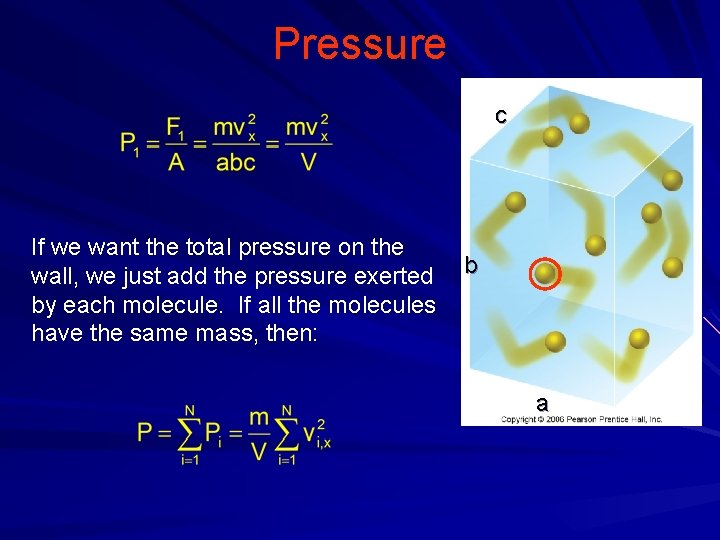
Pressure c If we want the total pressure on the wall, we just add the pressure exerted by each molecule. If all the molecules have the same mass, then: b a
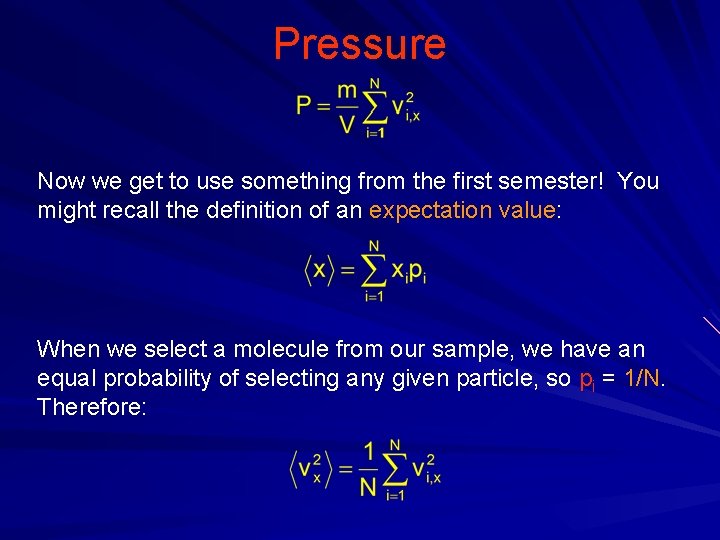
Pressure Now we get to use something from the first semester! You might recall the definition of an expectation value: When we select a molecule from our sample, we have an equal probability of selecting any given particle, so pi = 1/N. Therefore:
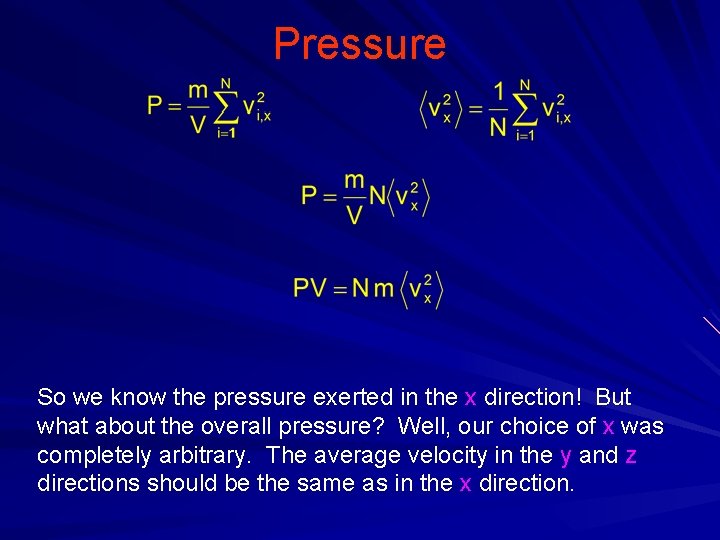
Pressure So we know the pressure exerted in the x direction! But what about the overall pressure? Well, our choice of x was completely arbitrary. The average velocity in the y and z directions should be the same as in the x direction.
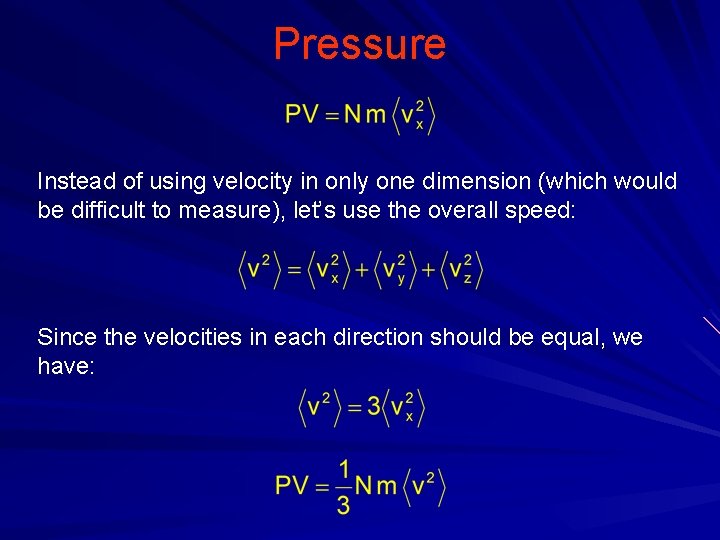
Pressure Instead of using velocity in only one dimension (which would be difficult to measure), let’s use the overall speed: Since the velocities in each direction should be equal, we have:
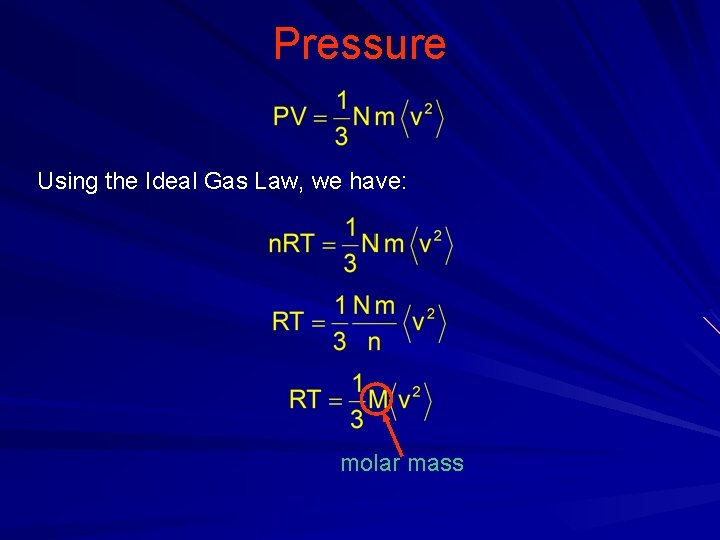
Pressure Using the Ideal Gas Law, we have: molar mass
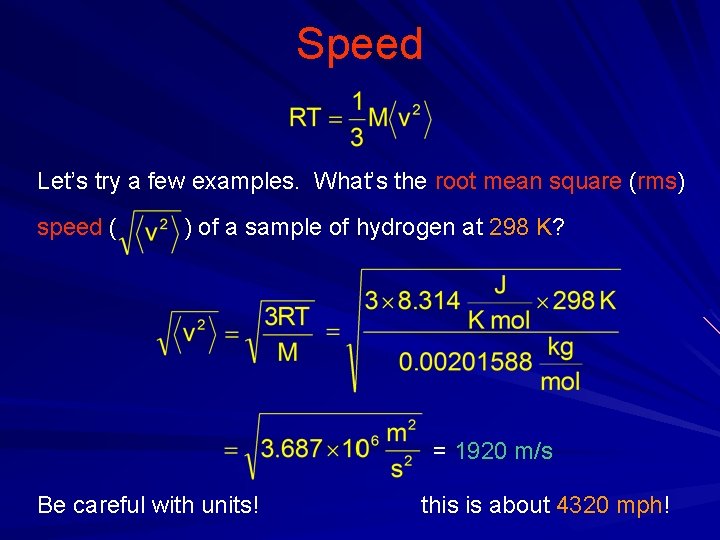
Speed Let’s try a few examples. What’s the root mean square (rms) speed ( ) of a sample of hydrogen at 298 K? = 1920 m/s Be careful with units! this is about 4320 mph!
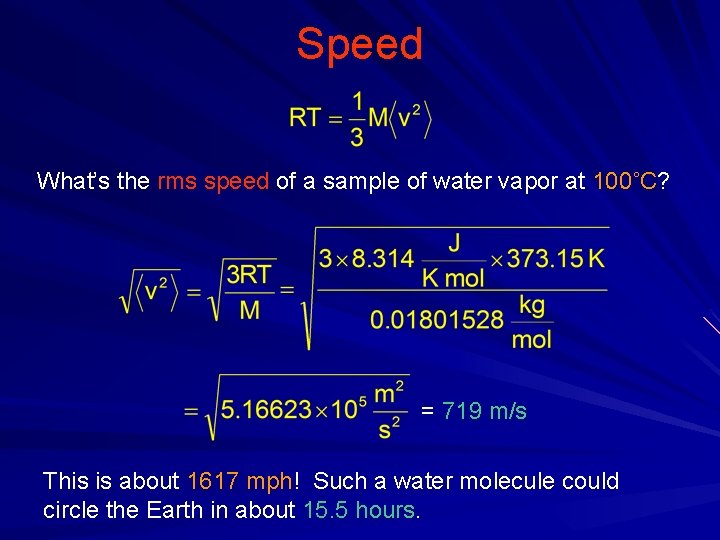
Speed What’s the rms speed of a sample of water vapor at 100˚C? = 719 m/s This is about 1617 mph! Such a water molecule could circle the Earth in about 15. 5 hours.
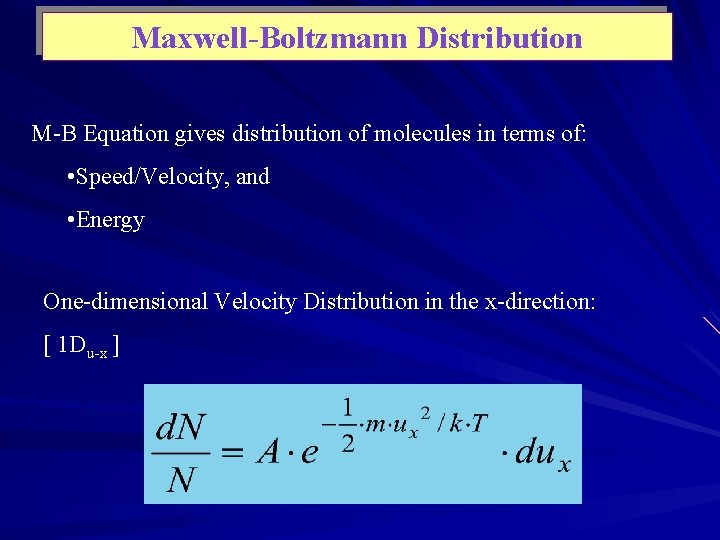
Maxwell-Boltzmann Distribution M-B Equation gives distribution of molecules in terms of: • Speed/Velocity, and • Energy One-dimensional Velocity Distribution in the x-direction: [ 1 Du-x ]
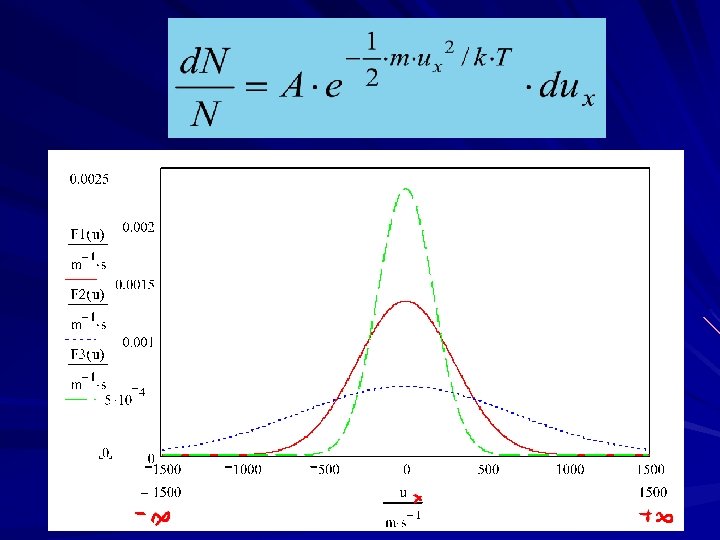
![3 D MaxwellBoltzmann Distribution 3 D Velocity Distribution Cartesian Coordinates 3 Du 3 D Maxwell-Boltzmann Distribution 3 D Velocity Distribution: Cartesian Coordinates: [ 3 Du ]](https://slidetodoc.com/presentation_image_h2/8561b2bf76979d16c978df483d4fe6d2/image-20.jpg)
3 D Maxwell-Boltzmann Distribution 3 D Velocity Distribution: Cartesian Coordinates: [ 3 Du ] , Let: a = m/2 k. T
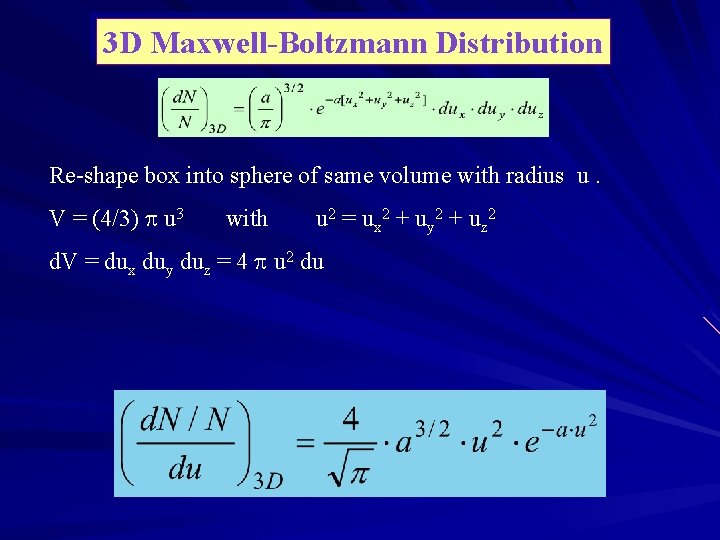
3 D Maxwell-Boltzmann Distribution Re-shape box into sphere of same volume with radius u. V = (4/3) u 3 with u 2 = u x 2 + u y 2 + u z 2 d. V = dux duy duz = 4 u 2 du
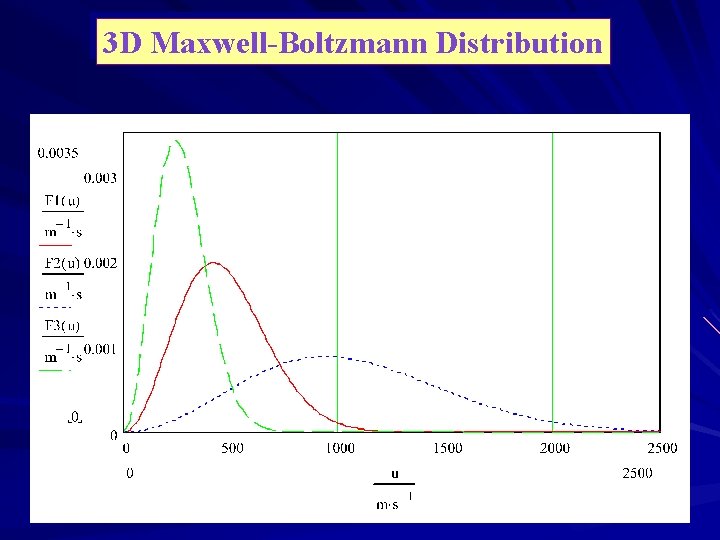
3 D Maxwell-Boltzmann Distribution Low T High T
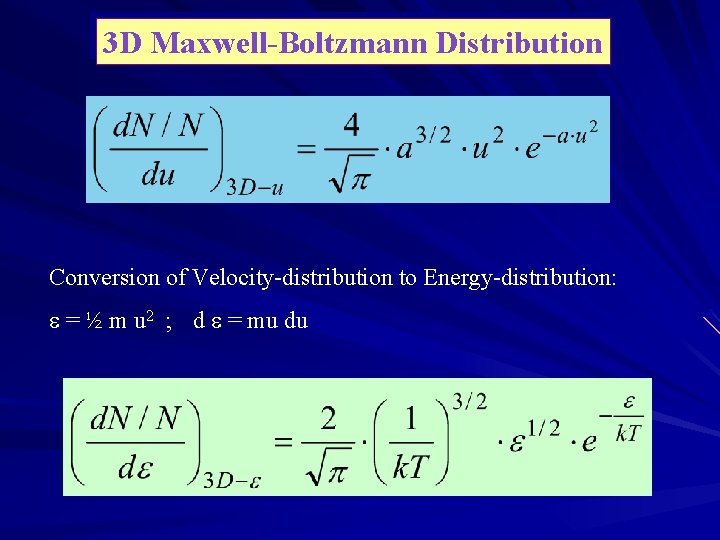
3 D Maxwell-Boltzmann Distribution Conversion of Velocity-distribution to Energy-distribution: = ½ m u 2 ; d = mu du
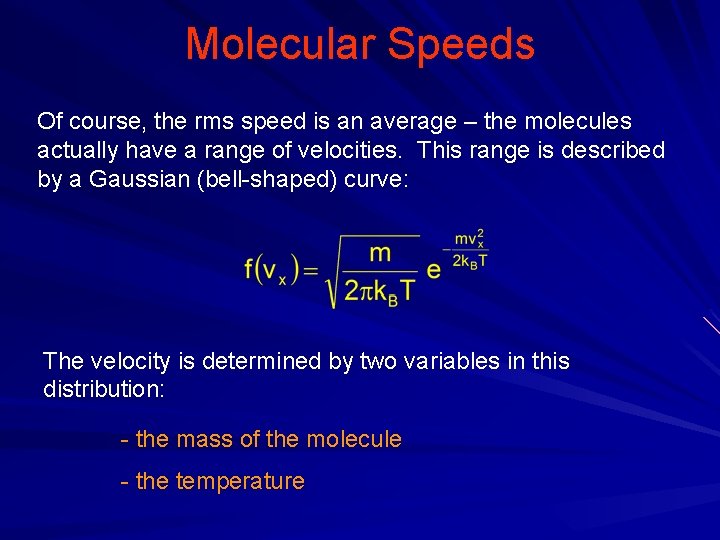
Molecular Speeds Of course, the rms speed is an average – the molecules actually have a range of velocities. This range is described by a Gaussian (bell-shaped) curve: The velocity is determined by two variables in this distribution: - the mass of the molecule - the temperature
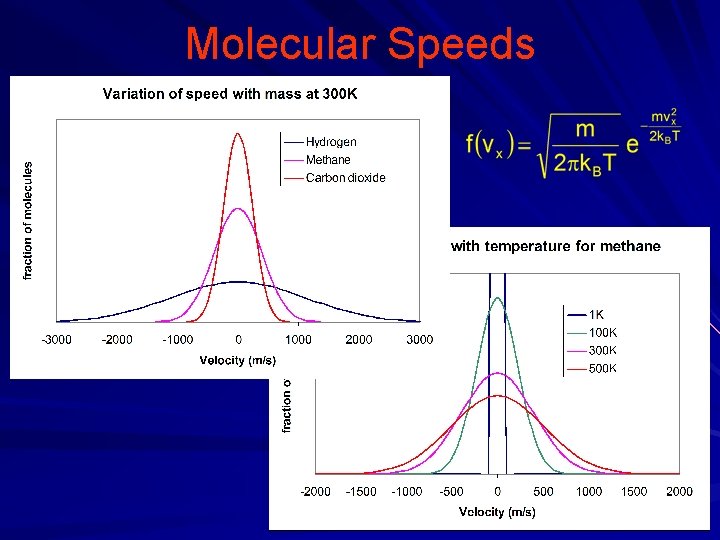
Molecular Speeds
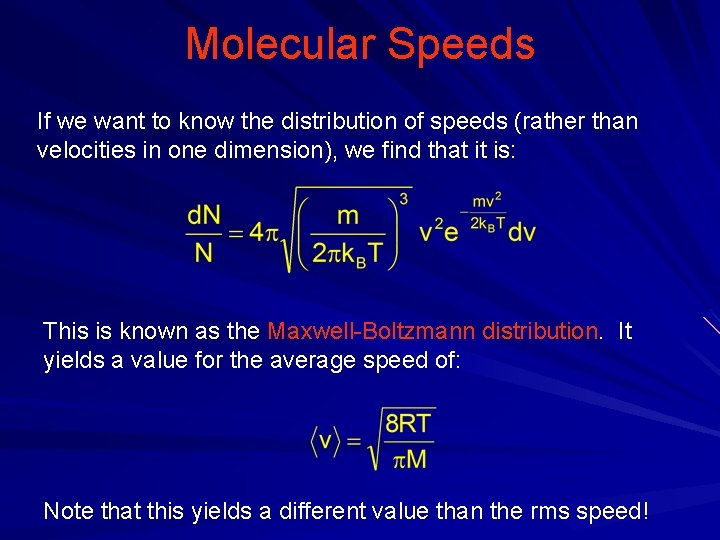
Molecular Speeds If we want to know the distribution of speeds (rather than velocities in one dimension), we find that it is: This is known as the Maxwell-Boltzmann distribution. It yields a value for the average speed of: Note that this yields a different value than the rms speed!
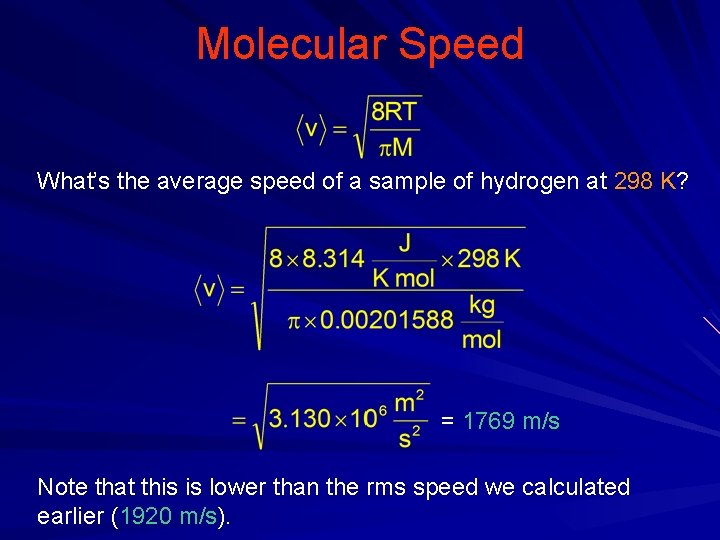
Molecular Speed What’s the average speed of a sample of hydrogen at 298 K? = 1769 m/s Note that this is lower than the rms speed we calculated earlier (1920 m/s).
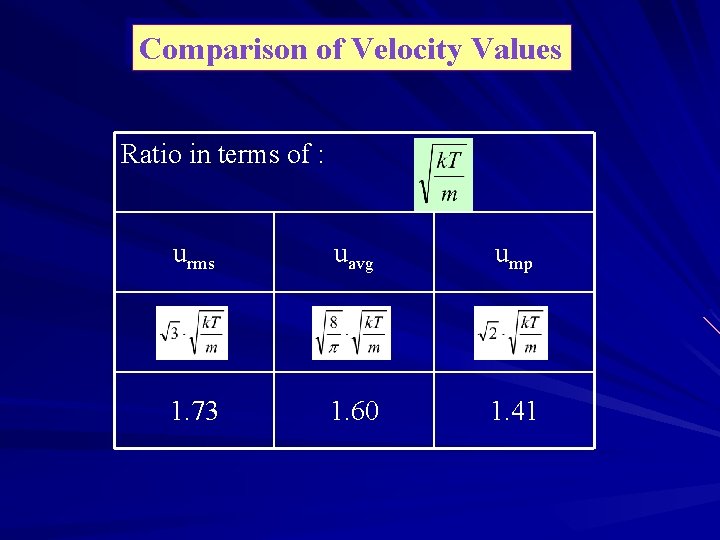
Comparison of Velocity Values Ratio in terms of : urms uavg ump 1. 73 1. 60 1. 41
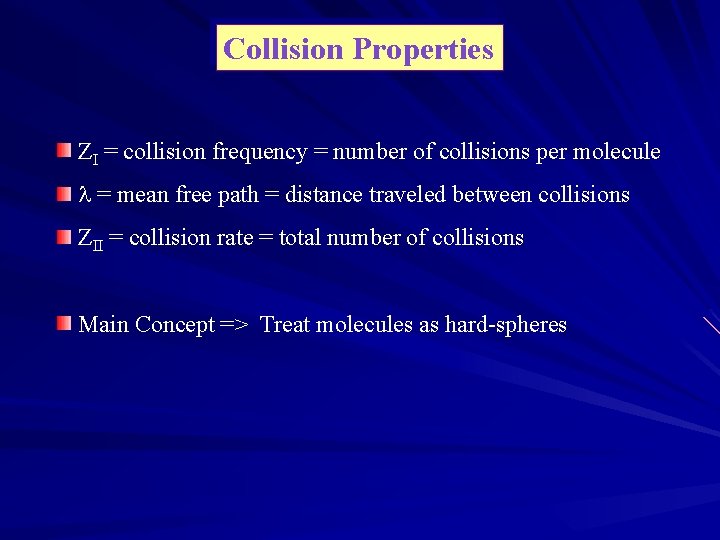
Collision Properties ZI = collision frequency = number of collisions per molecule = mean free path = distance traveled between collisions ZII = collision rate = total number of collisions Main Concept => Treat molecules as hard-spheres
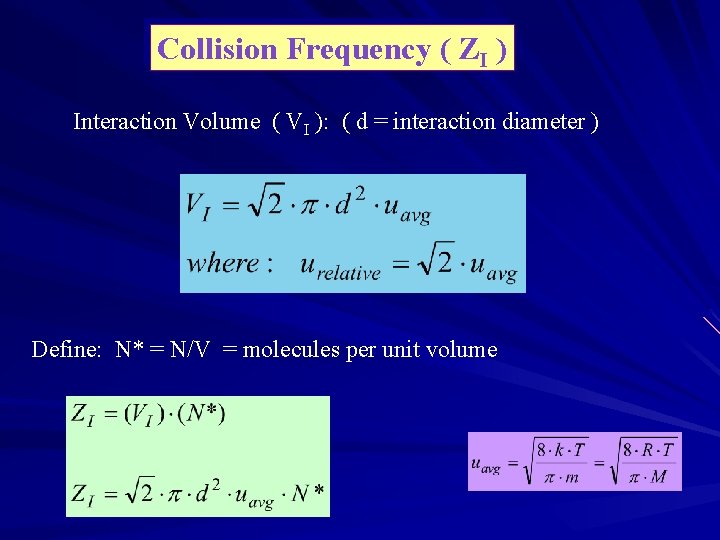
Collision Frequency ( ZI ) Interaction Volume ( VI ): ( d = interaction diameter ) Define: N* = N/V = molecules per unit volume
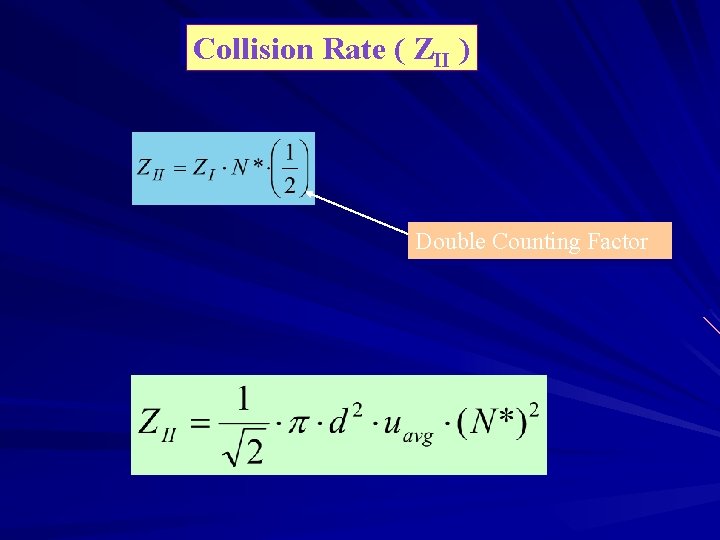
Collision Rate ( ZII ) Double Counting Factor
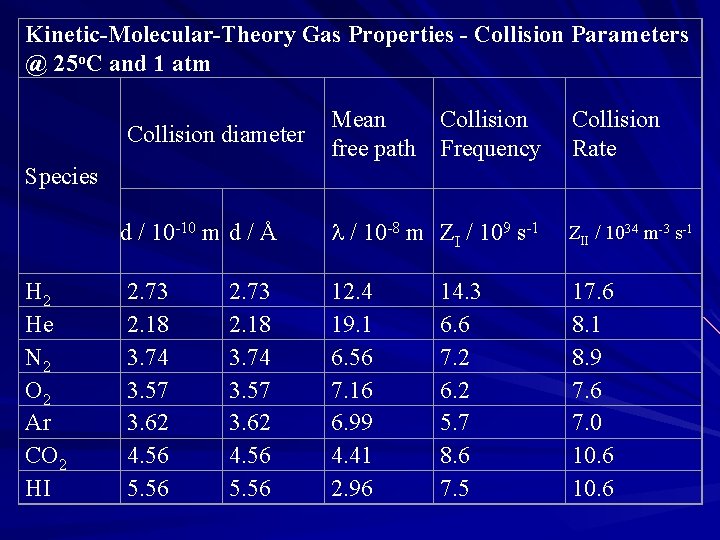
Kinetic-Molecular-Theory Gas Properties - Collision Parameters @ 25 o. C and 1 atm Collision diameter Mean free path Collision Frequency Collision Rate d / 10 -10 m d / Å / 10 -8 m ZI / 109 s-1 ZII / 1034 m-3 s-1 12. 4 19. 1 6. 56 7. 16 6. 99 4. 41 2. 96 17. 6 8. 1 8. 9 7. 6 7. 0 10. 6 Species H 2 He N 2 O 2 Ar CO 2 HI 2. 73 2. 18 3. 74 3. 57 3. 62 4. 56 5. 56 14. 3 6. 6 7. 2 6. 2 5. 7 8. 6 7. 5
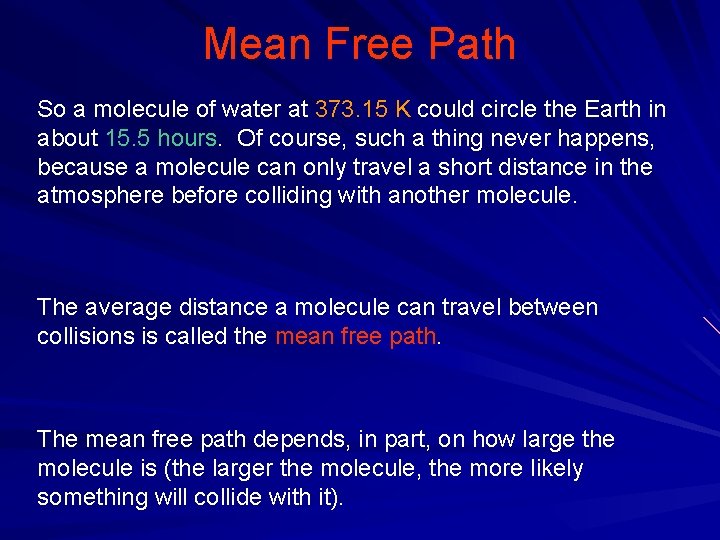
Mean Free Path So a molecule of water at 373. 15 K could circle the Earth in about 15. 5 hours. Of course, such a thing never happens, because a molecule can only travel a short distance in the atmosphere before colliding with another molecule. The average distance a molecule can travel between collisions is called the mean free path. The mean free path depends, in part, on how large the molecule is (the larger the molecule, the more likely something will collide with it).
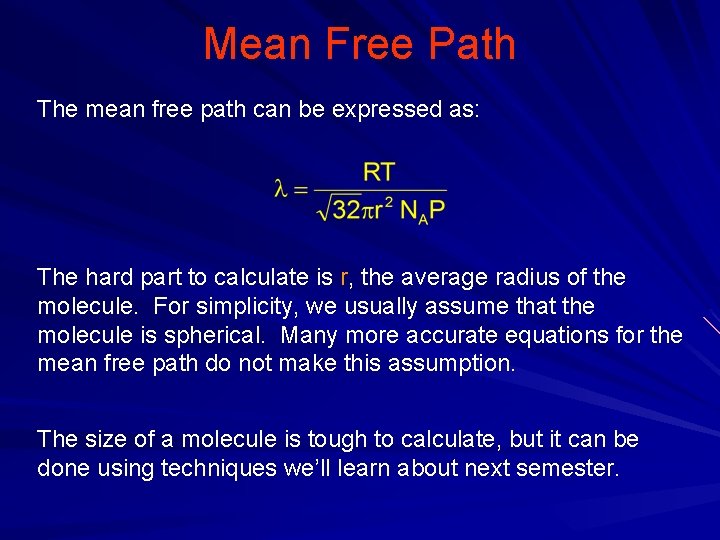
Mean Free Path The mean free path can be expressed as: The hard part to calculate is r, the average radius of the molecule. For simplicity, we usually assume that the molecule is spherical. Many more accurate equations for the mean free path do not make this assumption. The size of a molecule is tough to calculate, but it can be done using techniques we’ll learn about next semester.
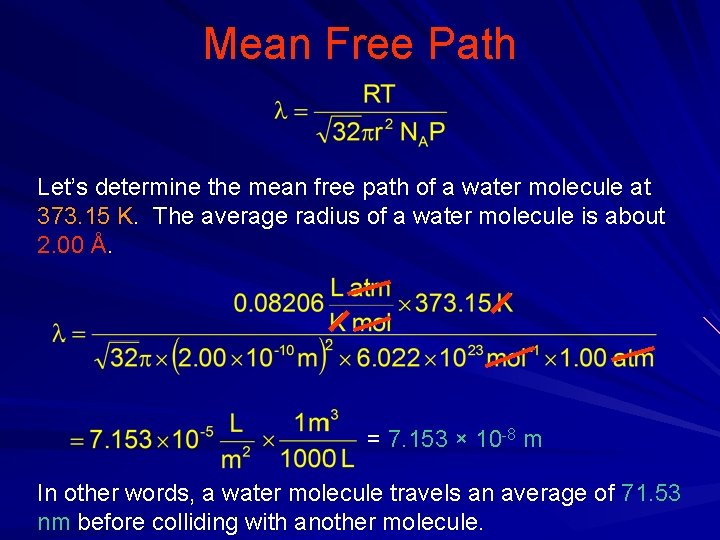
Mean Free Path Let’s determine the mean free path of a water molecule at 373. 15 K. The average radius of a water molecule is about 2. 00 Å. = 7. 153 × 10 -8 m In other words, a water molecule travels an average of 71. 53 nm before colliding with another molecule.
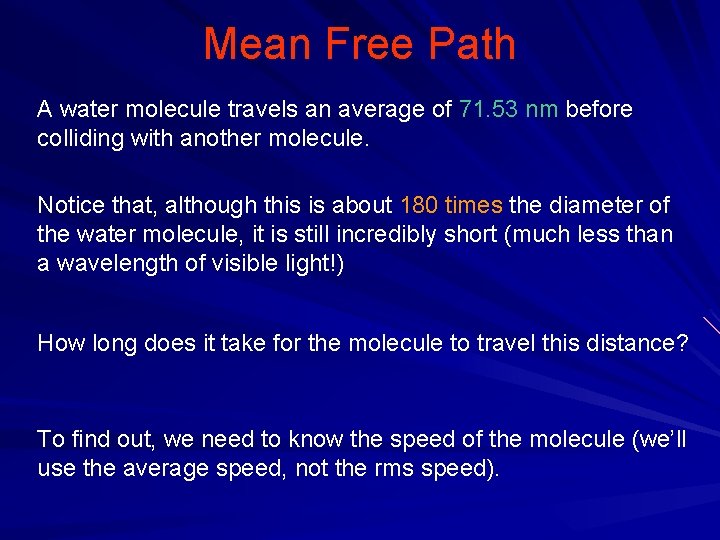
Mean Free Path A water molecule travels an average of 71. 53 nm before colliding with another molecule. Notice that, although this is about 180 times the diameter of the water molecule, it is still incredibly short (much less than a wavelength of visible light!) How long does it take for the molecule to travel this distance? To find out, we need to know the speed of the molecule (we’ll use the average speed, not the rms speed).
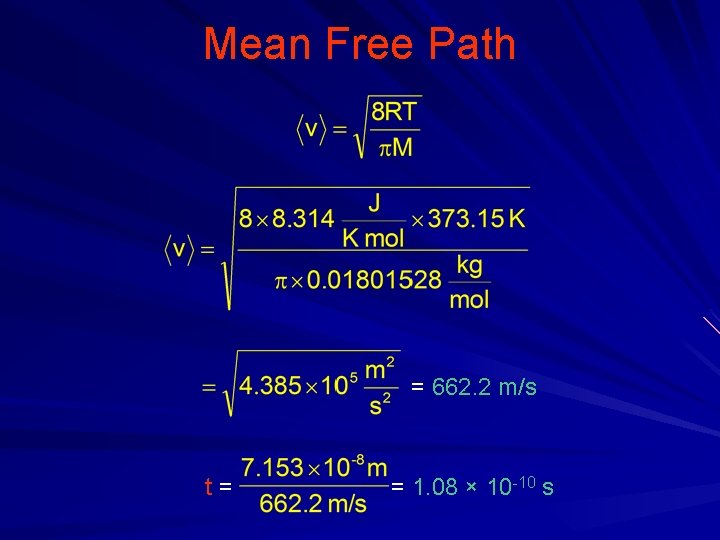
Mean Free Path = 662. 2 m/s t= = 1. 08 × 10 -10 s
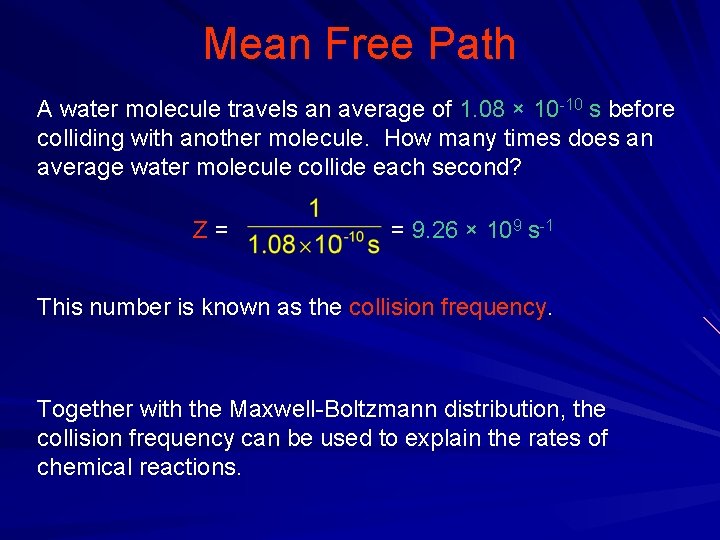
Mean Free Path A water molecule travels an average of 1. 08 × 10 -10 s before colliding with another molecule. How many times does an average water molecule collide each second? Z= = 9. 26 × 109 s-1 This number is known as the collision frequency. Together with the Maxwell-Boltzmann distribution, the collision frequency can be used to explain the rates of chemical reactions.
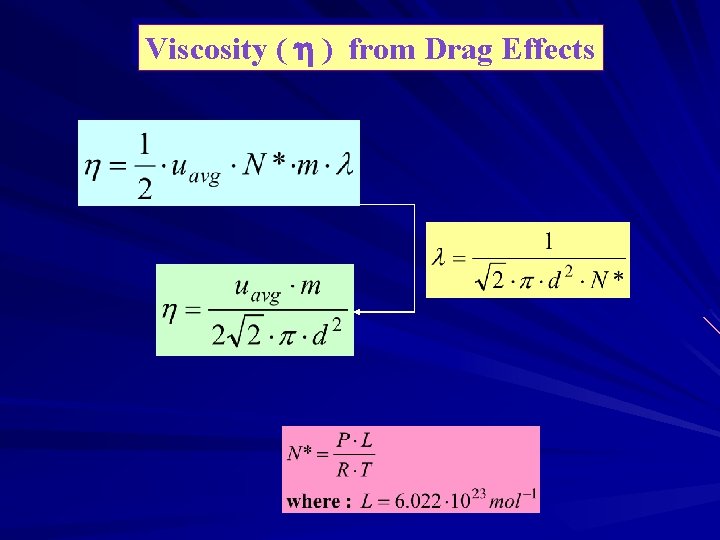
Viscosity ( ) from Drag Effects
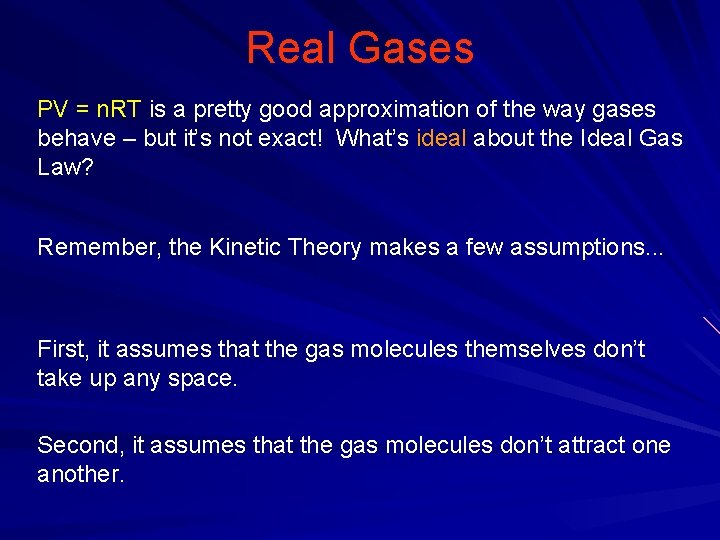
Real Gases PV = n. RT is a pretty good approximation of the way gases behave – but it’s not exact! What’s ideal about the Ideal Gas Law? Remember, the Kinetic Theory makes a few assumptions. . . First, it assumes that the gas molecules themselves don’t take up any space. Second, it assumes that the gas molecules don’t attract one another.
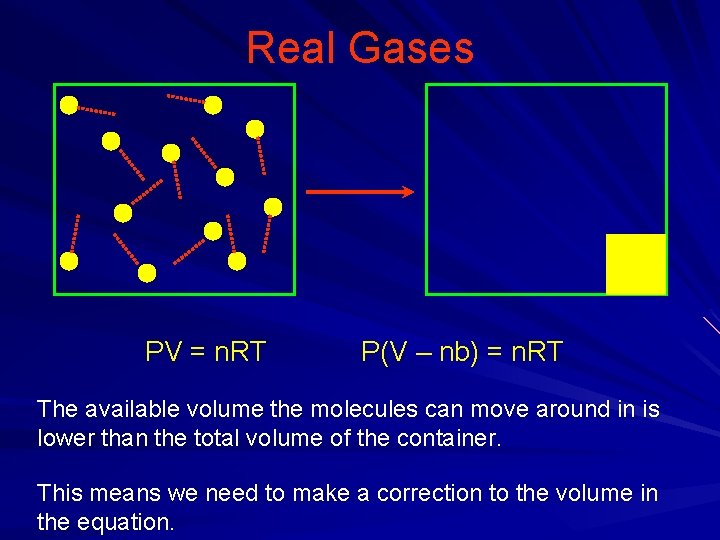
Real Gases PV = n. RT P(V – nb) = n. RT The available volume the molecules can move around in is lower than the total volume of the container. This means we need to make a correction to the volume in the equation.
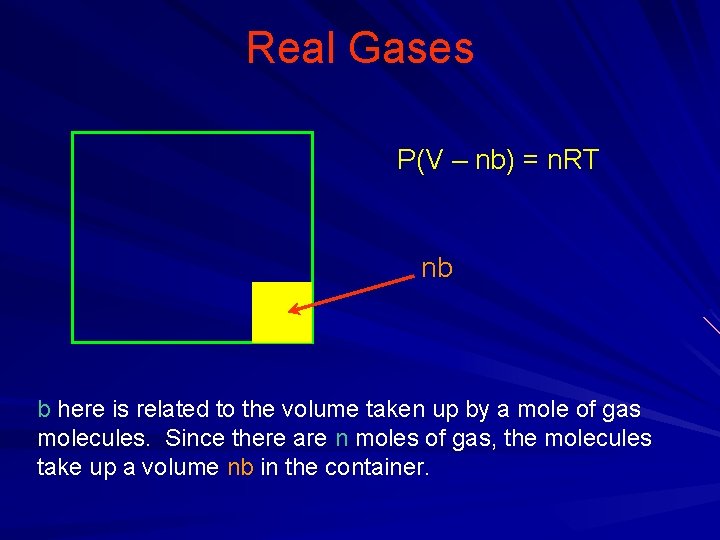
Real Gases P(V – nb) = n. RT nb b here is related to the volume taken up by a mole of gas molecules. Since there are n moles of gas, the molecules take up a volume nb in the container.
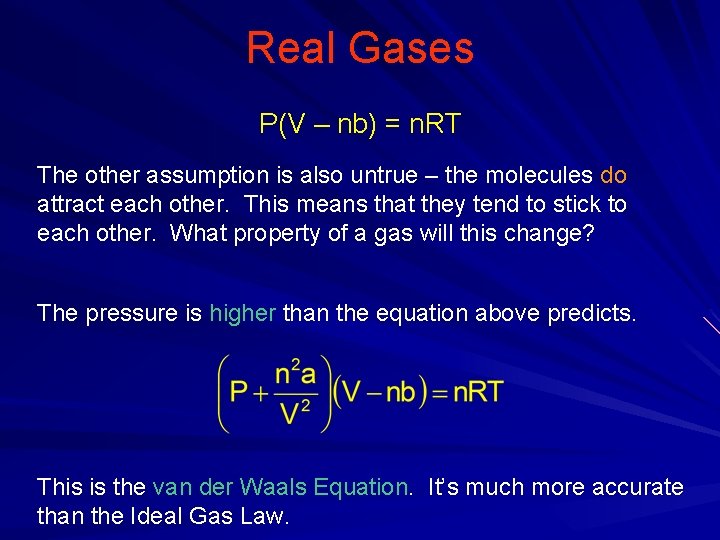
Real Gases P(V – nb) = n. RT The other assumption is also untrue – the molecules do attract each other. This means that they tend to stick to each other. What property of a gas will this change? The pressure is higher than the equation above predicts. This is the van der Waals Equation. It’s much more accurate than the Ideal Gas Law.
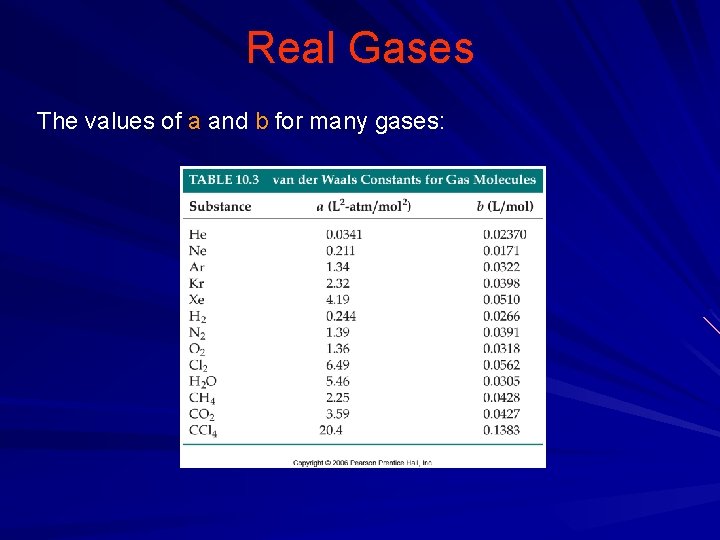
Real Gases The values of a and b for many gases:
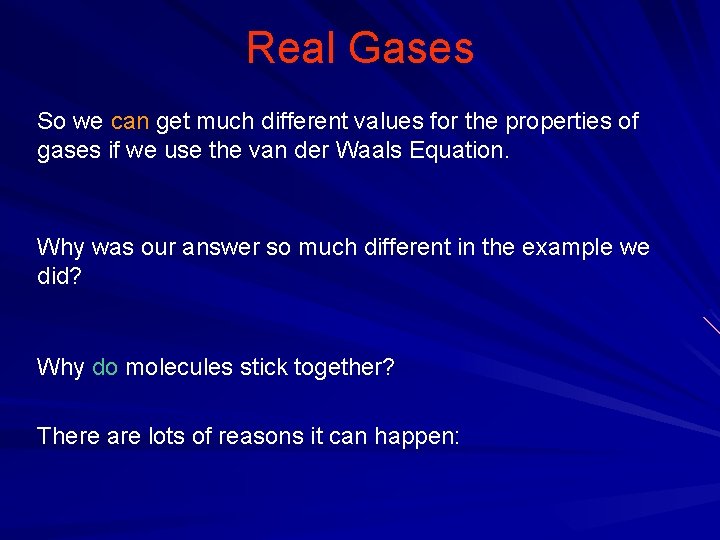
Real Gases So we can get much different values for the properties of gases if we use the van der Waals Equation. Why was our answer so much different in the example we did? Why do molecules stick together? There are lots of reasons it can happen:
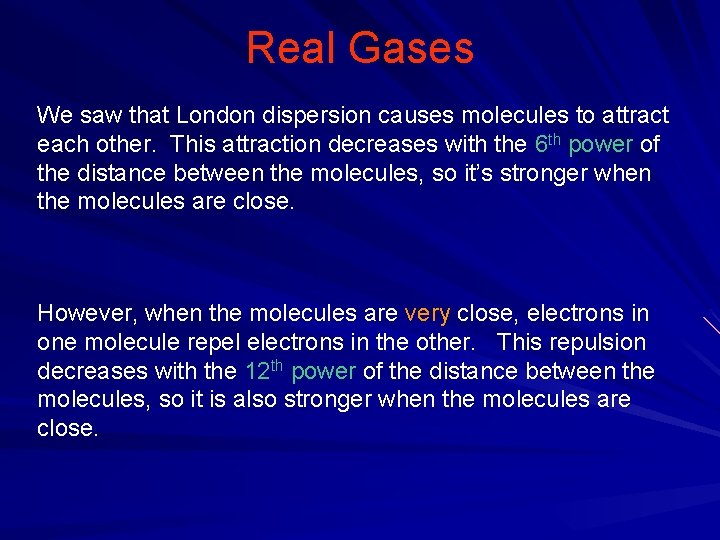
Real Gases We saw that London dispersion causes molecules to attract each other. This attraction decreases with the 6 th power of the distance between the molecules, so it’s stronger when the molecules are close. However, when the molecules are very close, electrons in one molecule repel electrons in the other. This repulsion decreases with the 12 th power of the distance between the molecules, so it is also stronger when the molecules are close.
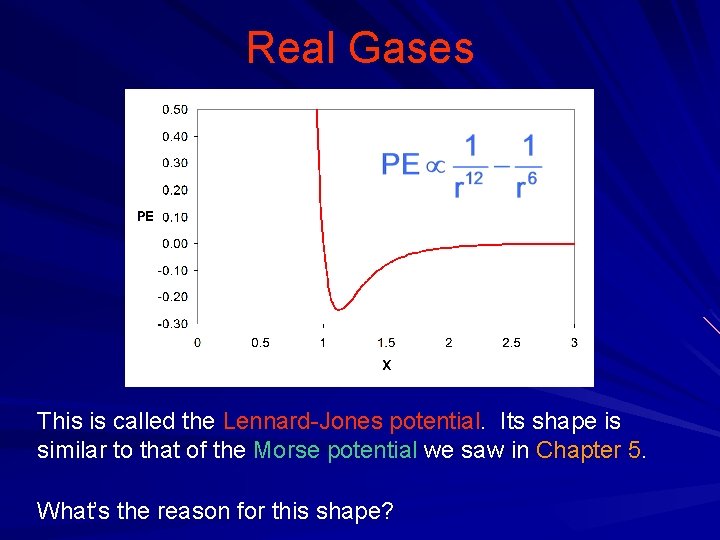
Real Gases This is called the Lennard-Jones potential. Its shape is similar to that of the Morse potential we saw in Chapter 5. What’s the reason for this shape?
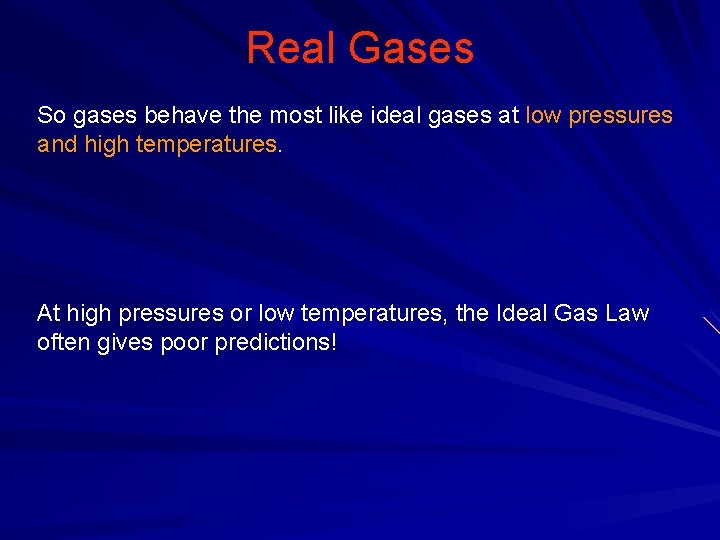
Real Gases So gases behave the most like ideal gases at low pressures and high temperatures. At high pressures or low temperatures, the Ideal Gas Law often gives poor predictions!
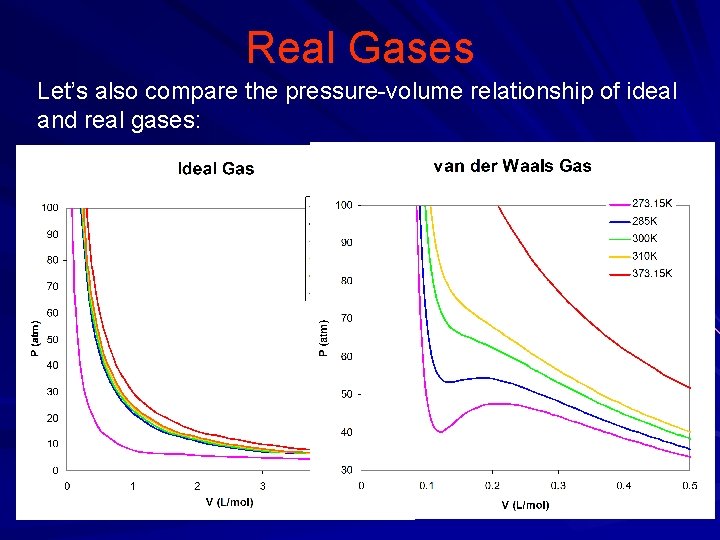
Real Gases Let’s also compare the pressure-volume relationship of ideal and real gases:
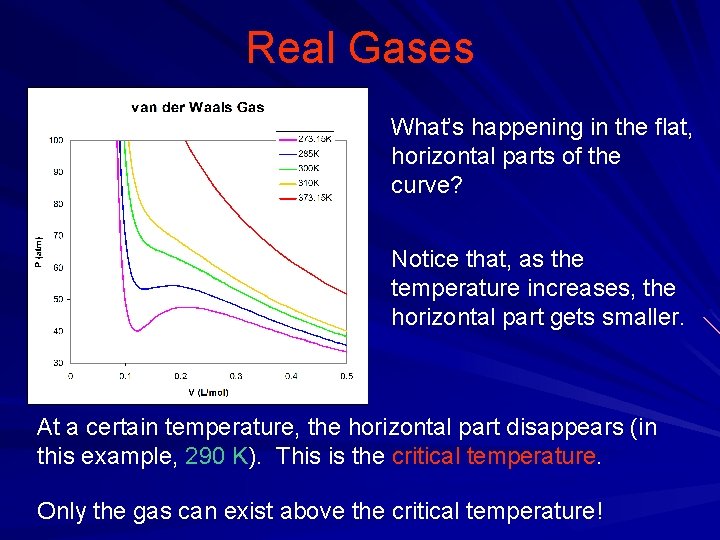
Real Gases What’s happening in the flat, horizontal parts of the curve? Notice that, as the temperature increases, the horizontal part gets smaller. At a certain temperature, the horizontal part disappears (in this example, 290 K). This is the critical temperature. Only the gas can exist above the critical temperature!
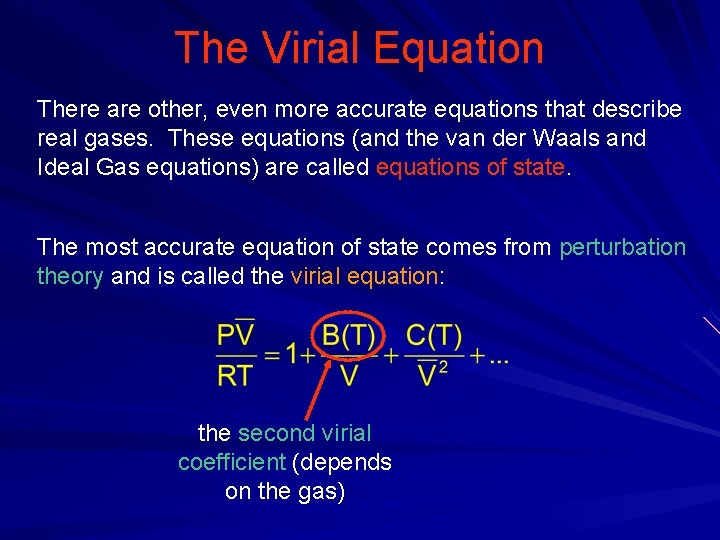
The Virial Equation There are other, even more accurate equations that describe real gases. These equations (and the van der Waals and Ideal Gas equations) are called equations of state. The most accurate equation of state comes from perturbation theory and is called the virial equation: the second virial coefficient (depends on the gas)
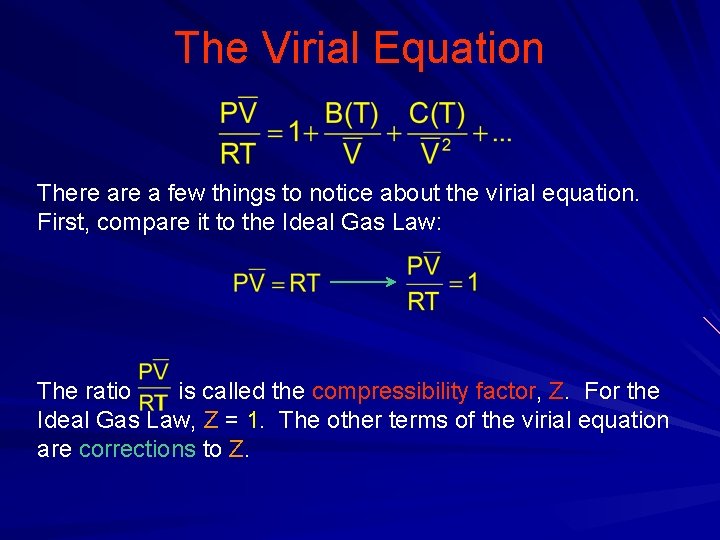
The Virial Equation There a few things to notice about the virial equation. First, compare it to the Ideal Gas Law: The ratio is called the compressibility factor, Z. For the Ideal Gas Law, Z = 1. The other terms of the virial equation are corrections to Z.
Characteristics of sanitary well
Eat well stay well
Diet for spinal cord injury patient
The part can never be well unless the whole is well
A collection of a well-defined objects
"to be happy is to live well and to do well."
How to write a conclusio
A combination of physical, mental, and social well-being.
A combination of physical, mental, and social well-being.
Health is the state of complete physical
Health is state of complete physical
Why does it happen
University physics with modern physics fifteenth edition
Physics ia ideas mechanics
General physics chapters
General physics 1 measurements
General physics halliday
Phy 1214
Applications of magnetism
General physics
Acceleration definition
General physics
Planos en cinematografia
Where did general lee surrender to general grant?
Units physical quantities and measurements
Physics and chemistry 4 eso
A person screaming
Neonatal jaundice physical examination
Assisting with a general physical examination
General physical examination
General appearence
General chemistry with qualitative analysis
General chemistry thermochemistry
General chemistry nomenclature
General chemistry 2
Klorpentan
General chemistry 11th edition
General chemistry 1 stoichiometry
General chemistry
General chemistry
General chemistry
General chemistry
General chemistry
Gasses
Physical and non-physical rate fences
Physical fitness test grade 9
What is rheochor in physical chemistry
Physical setting chemistry
Physical chemistry crash course
Hittorf theoretical device
Physical chemistry problems
Scope of physical chemistry
What are the chemical change