Crystal Structures 1 The Solid State Four state
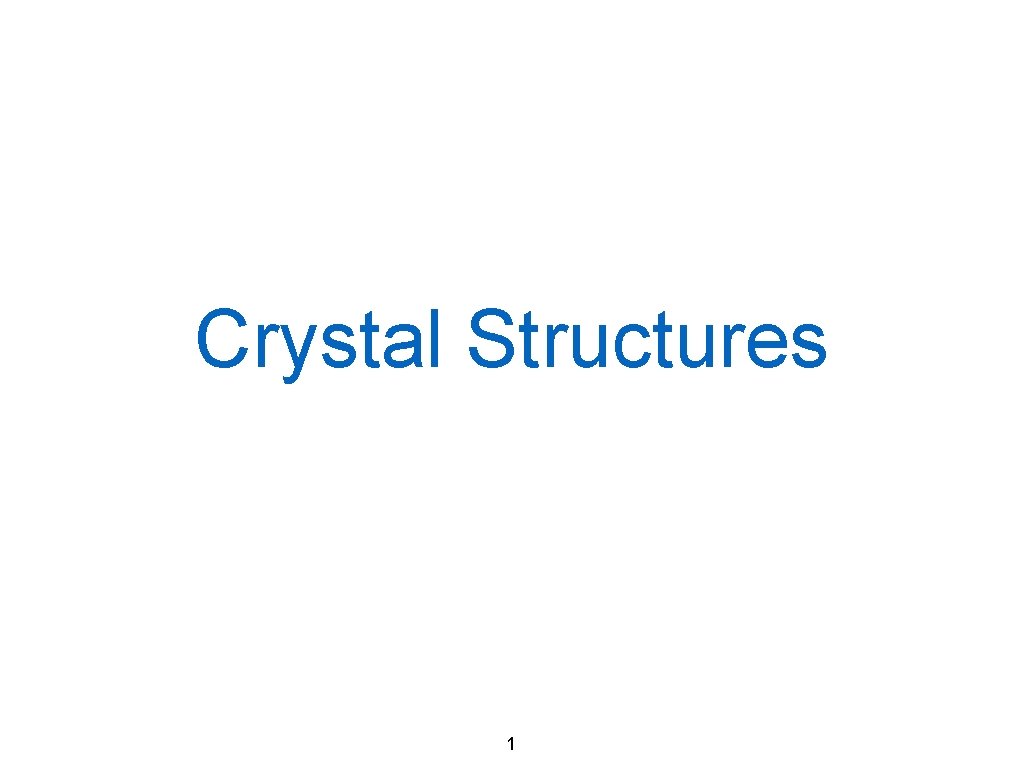
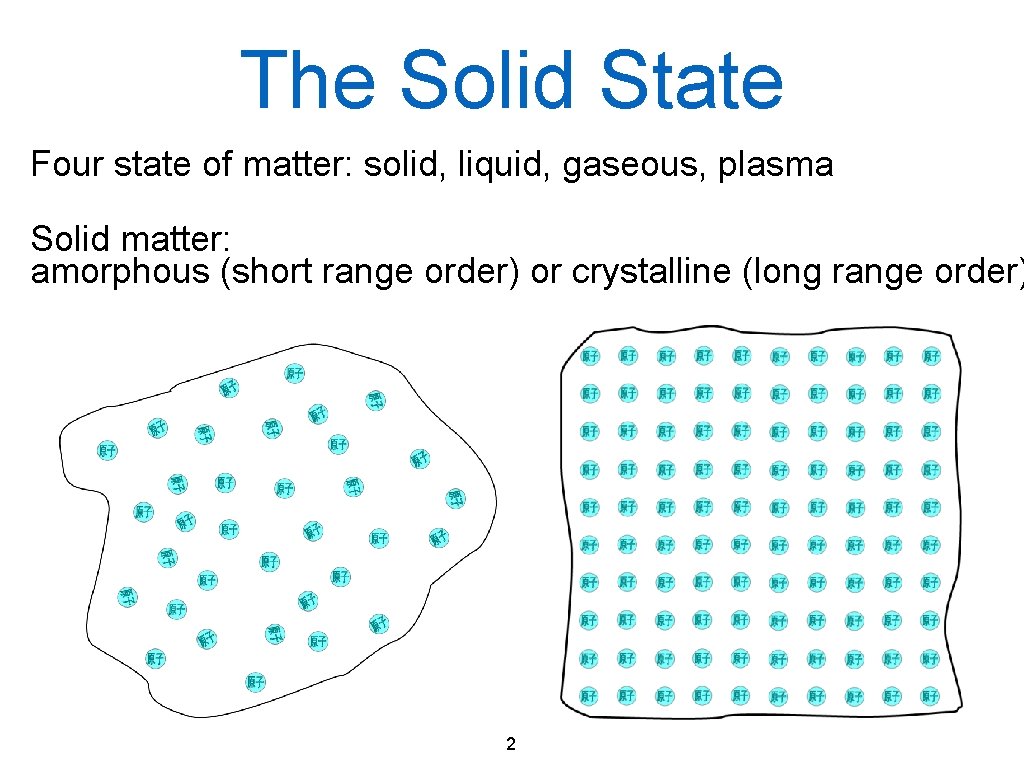
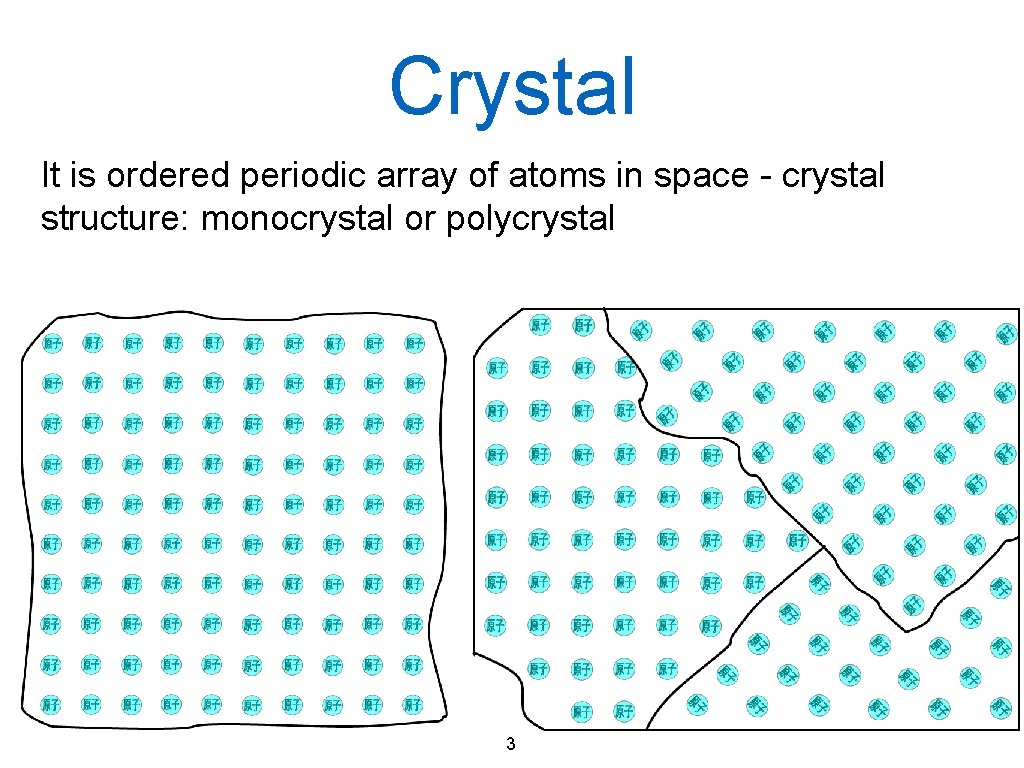
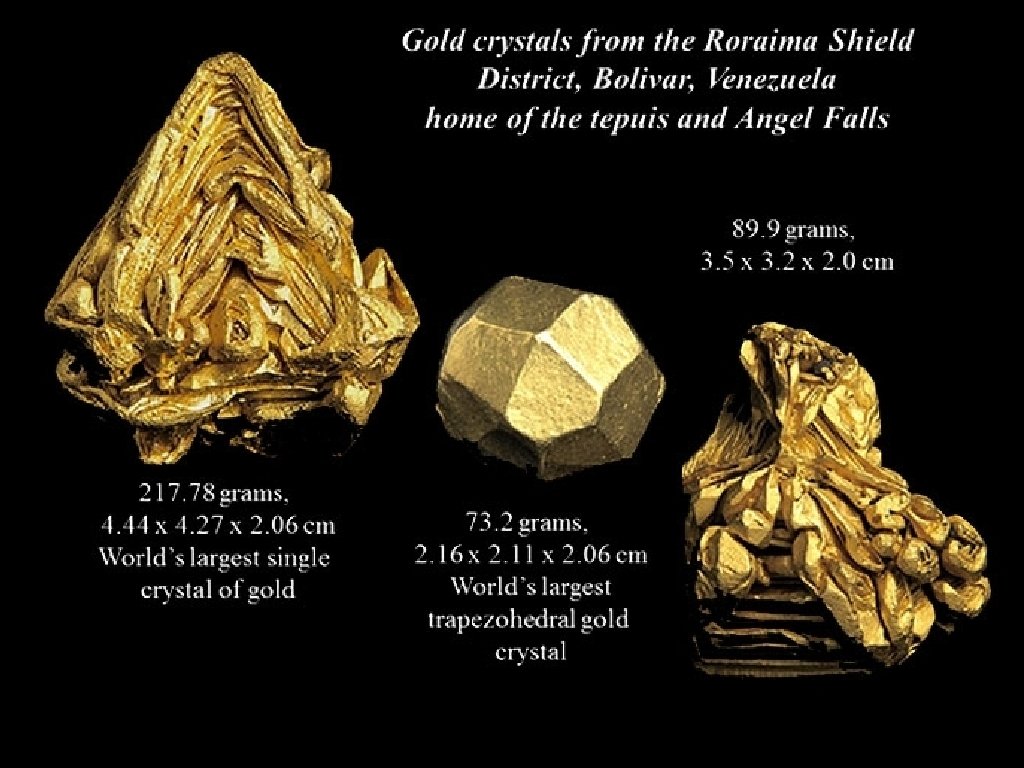
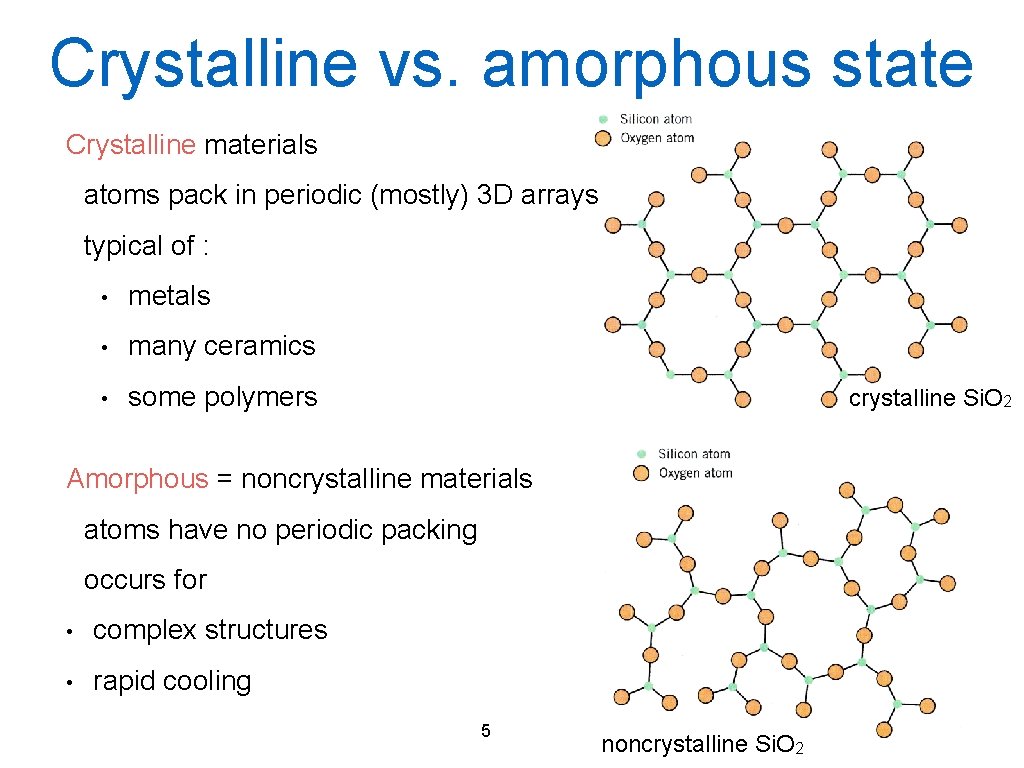
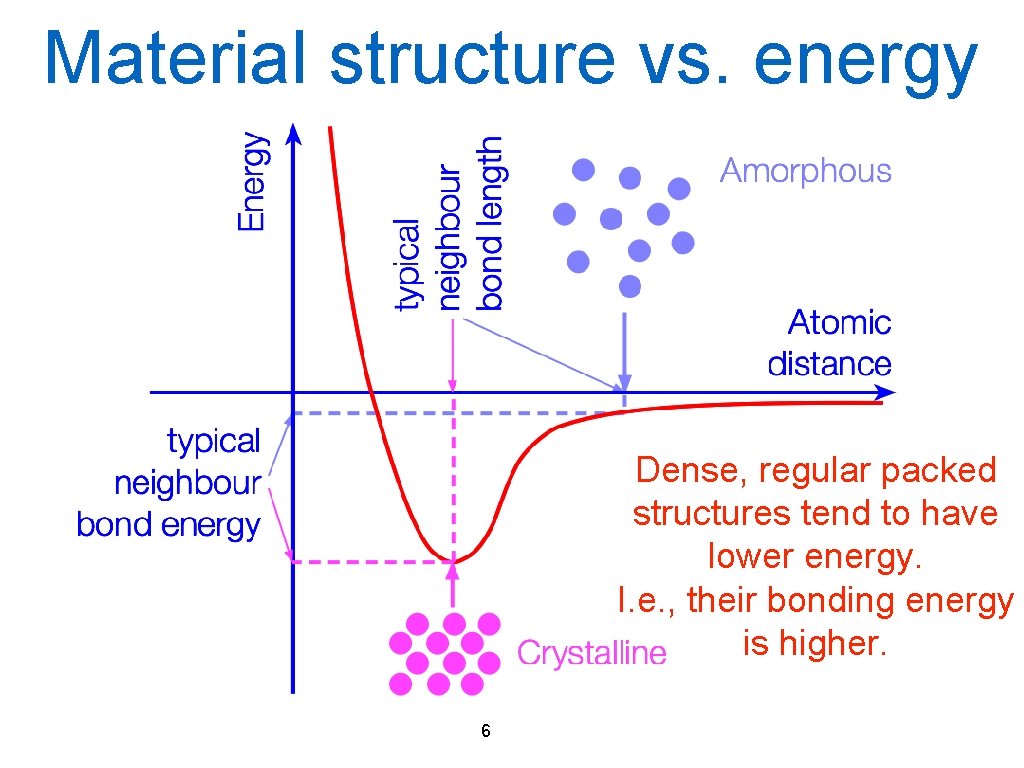
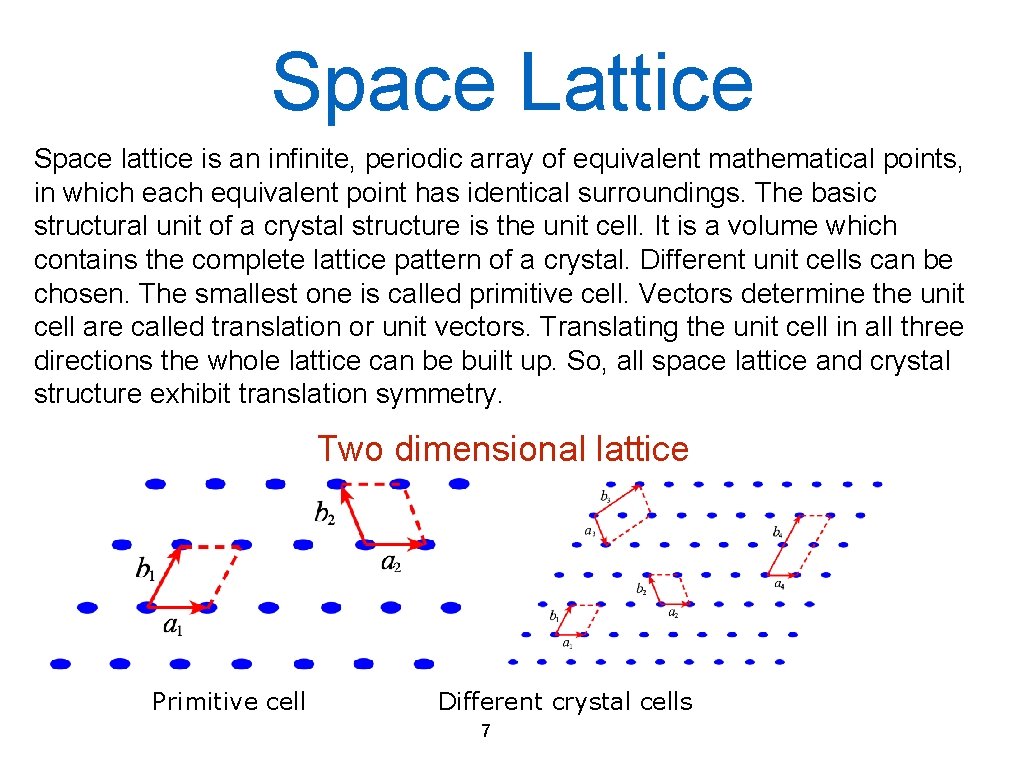
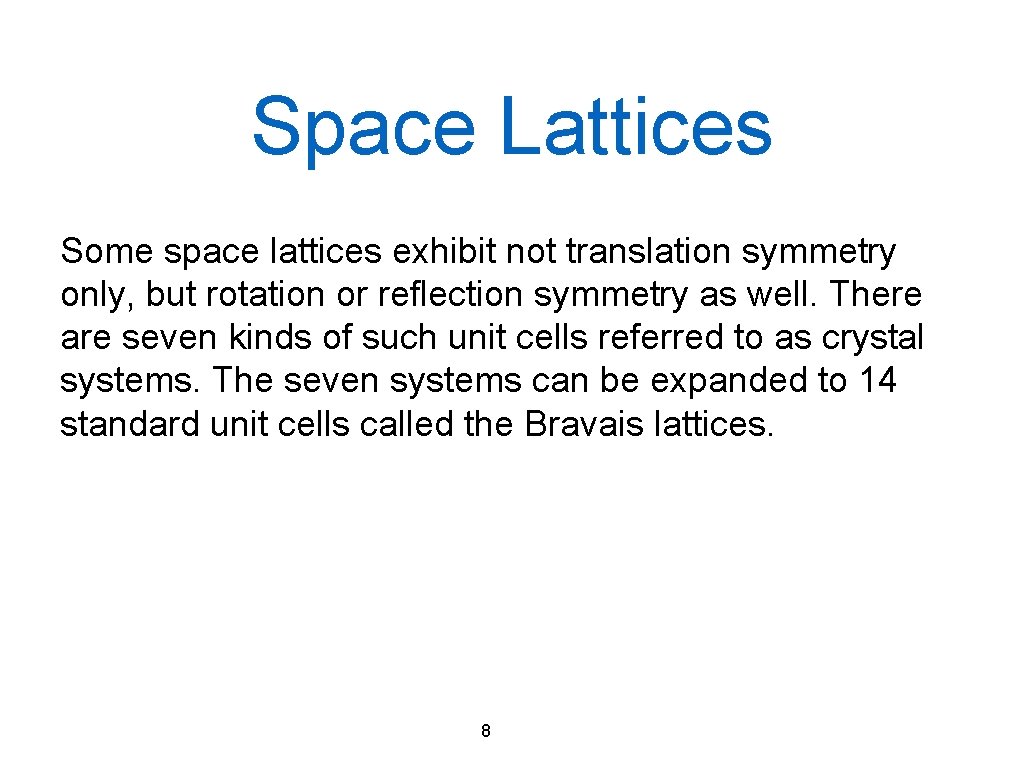
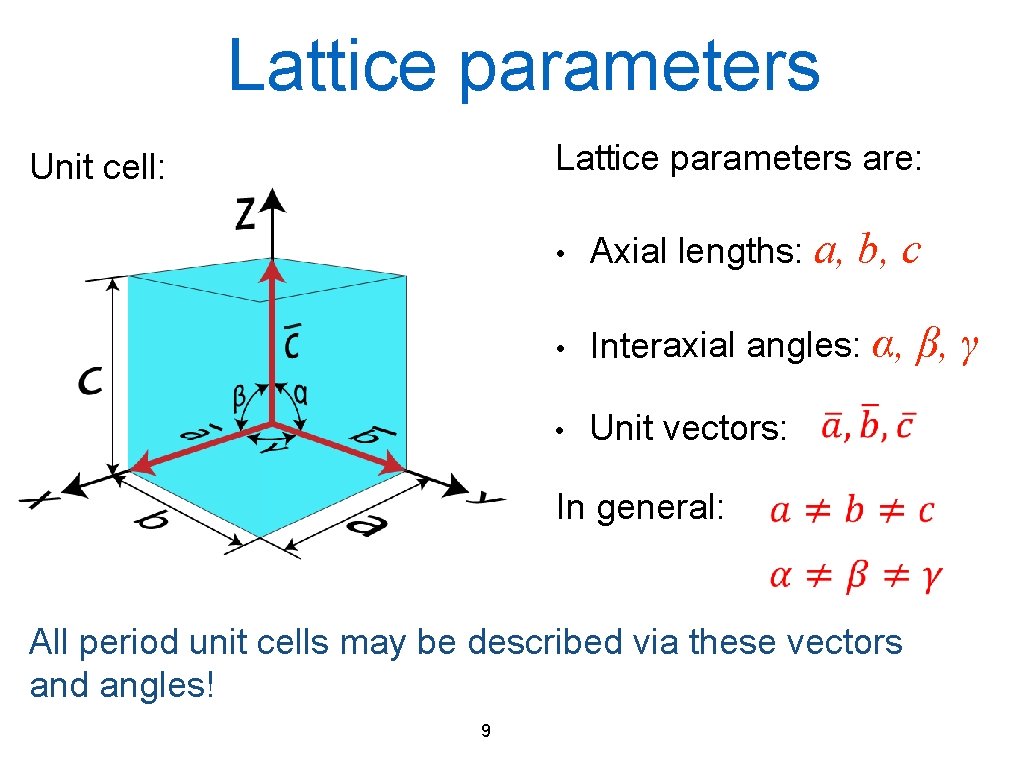
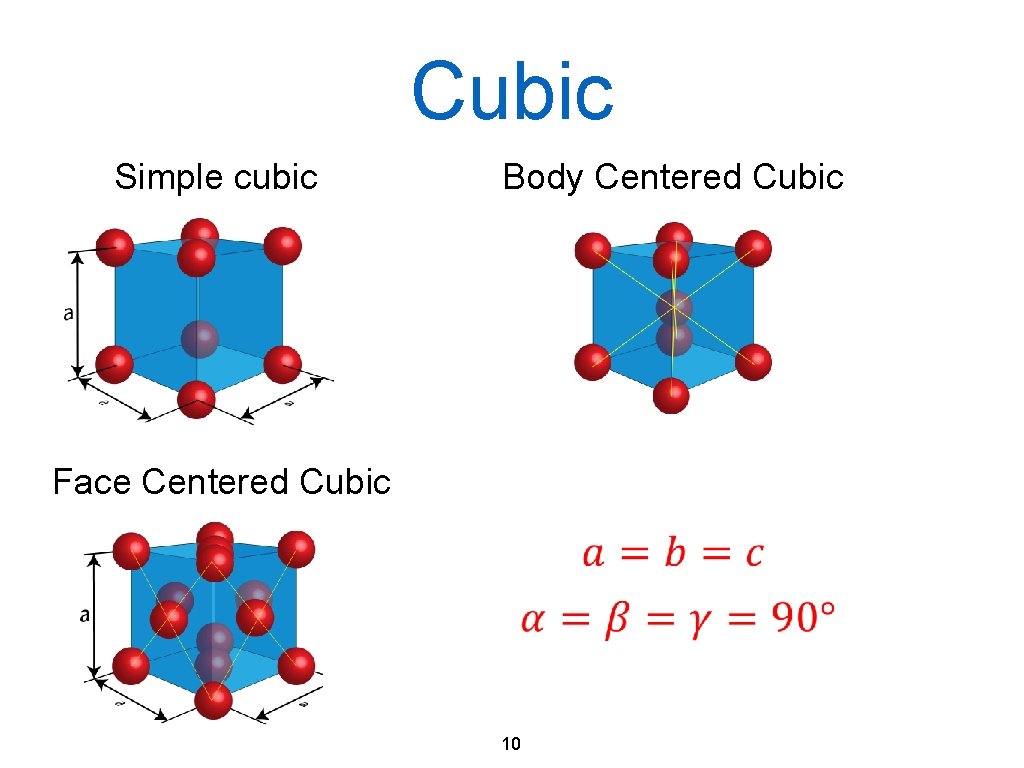
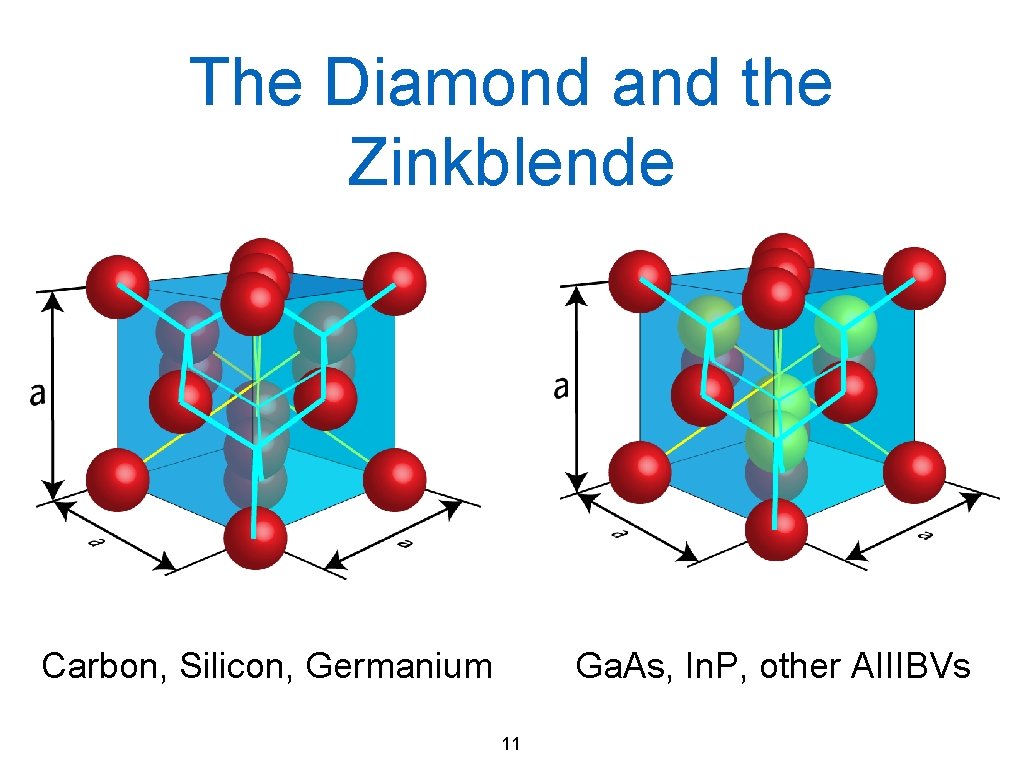
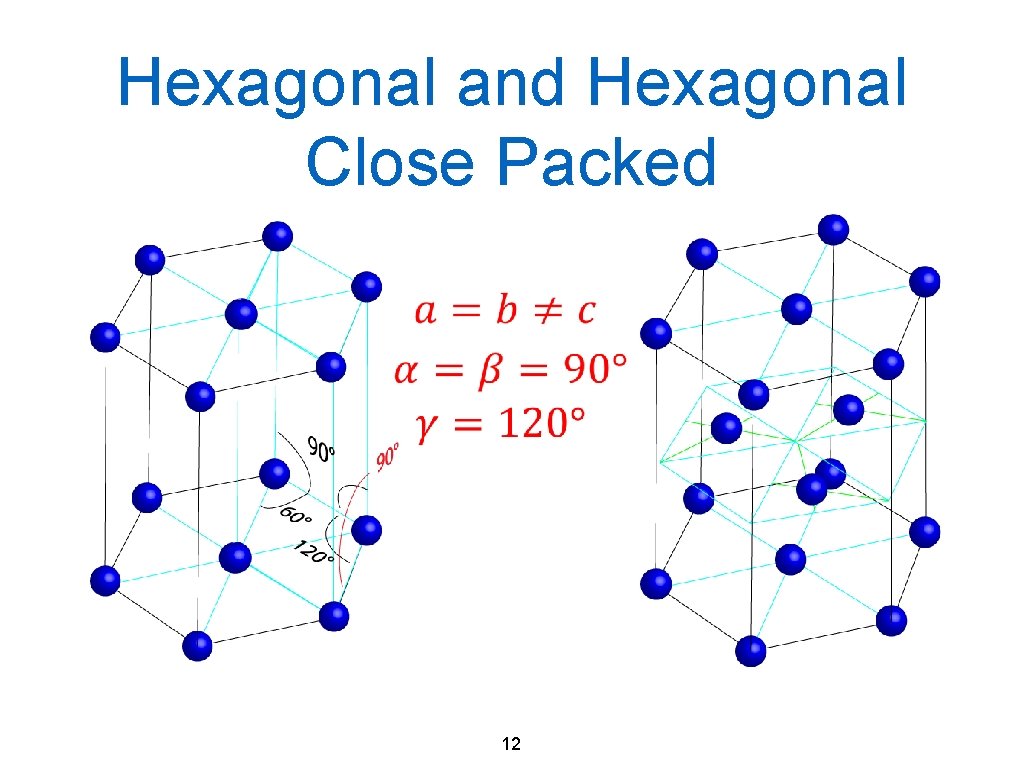
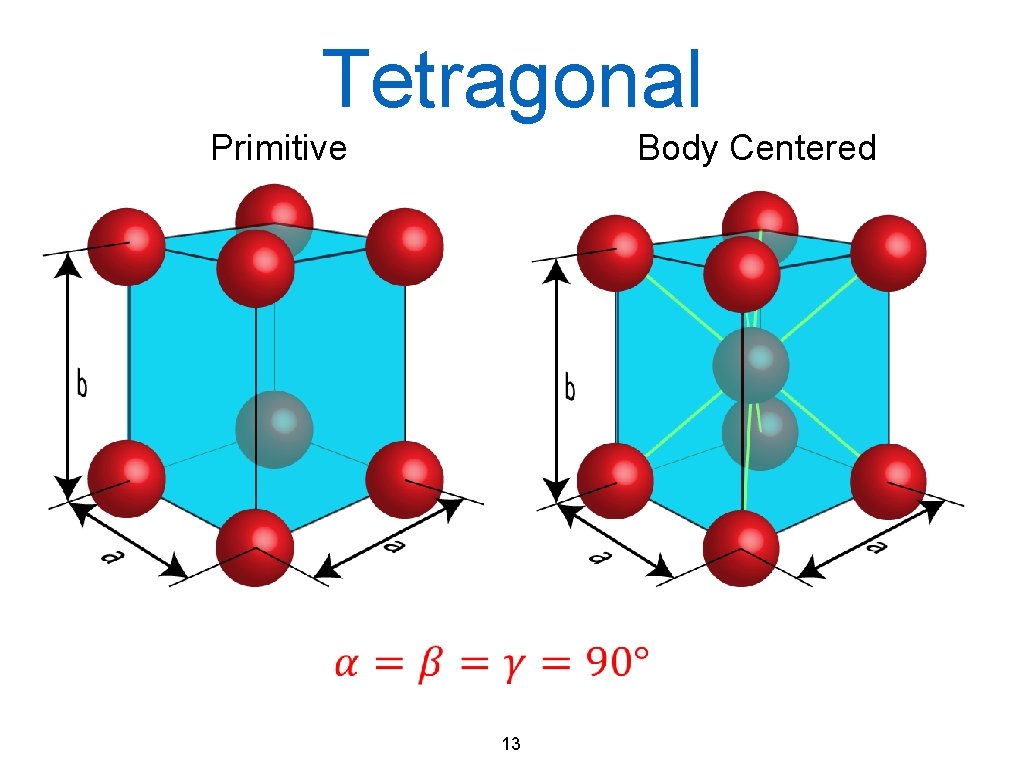
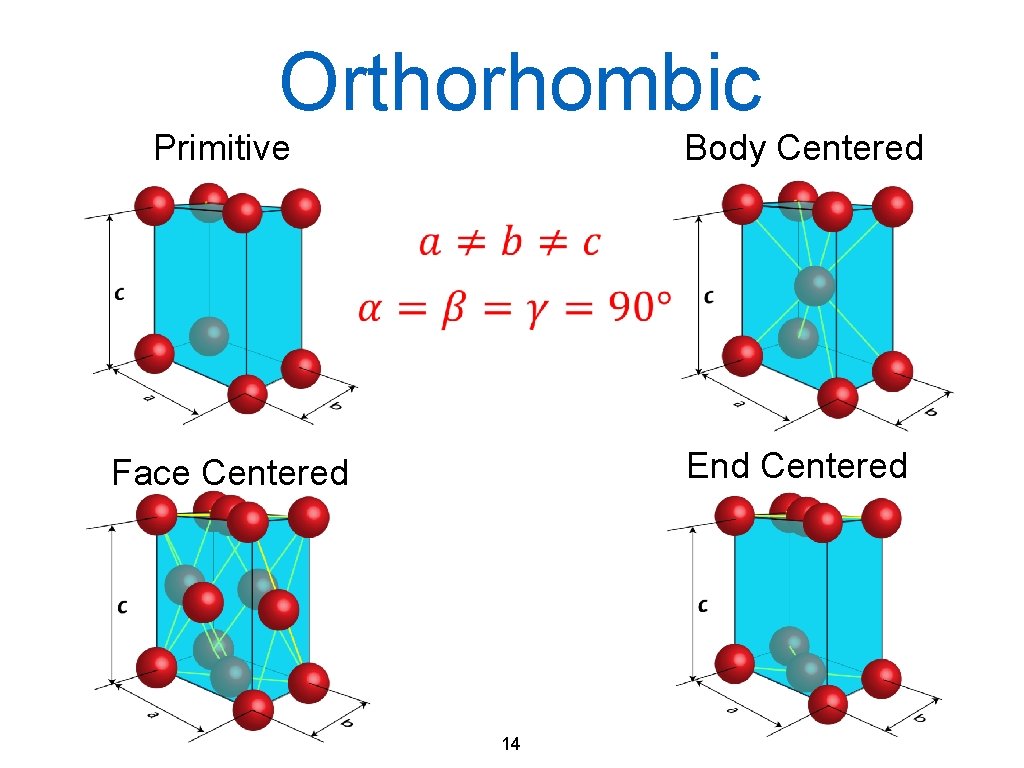
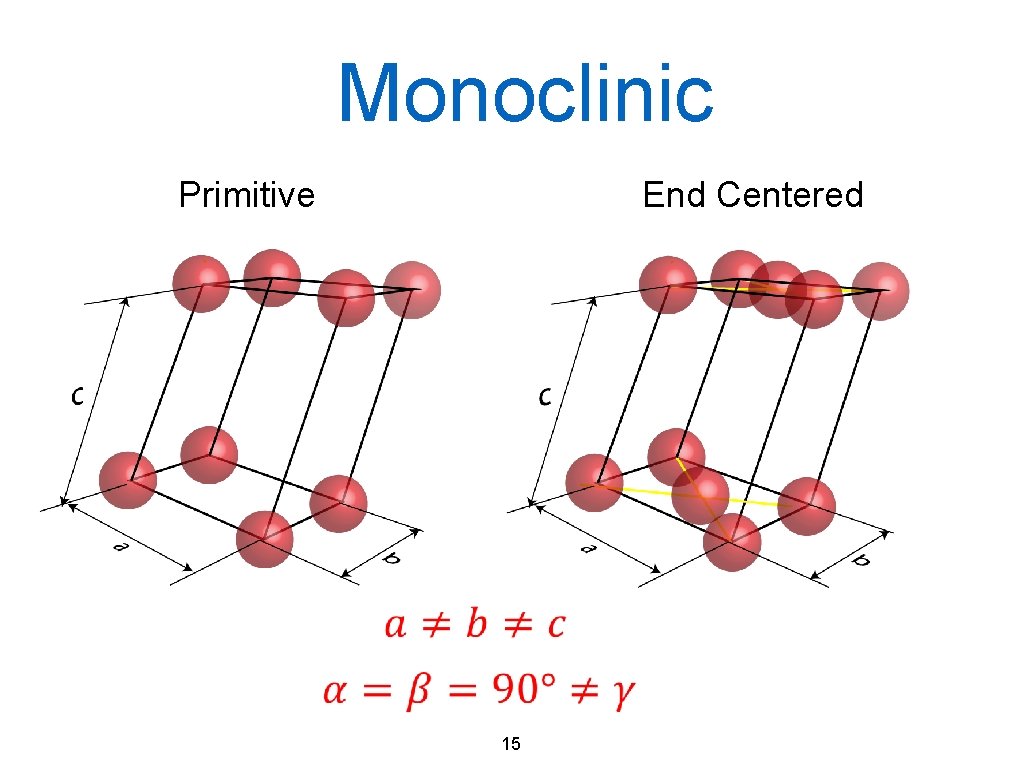
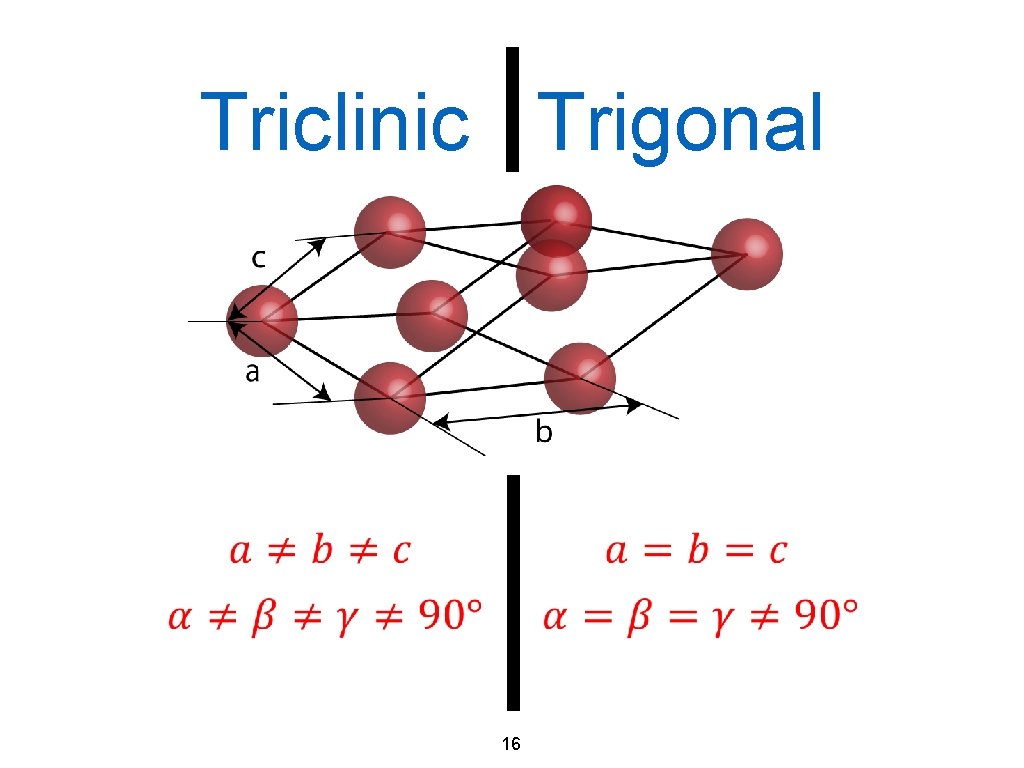
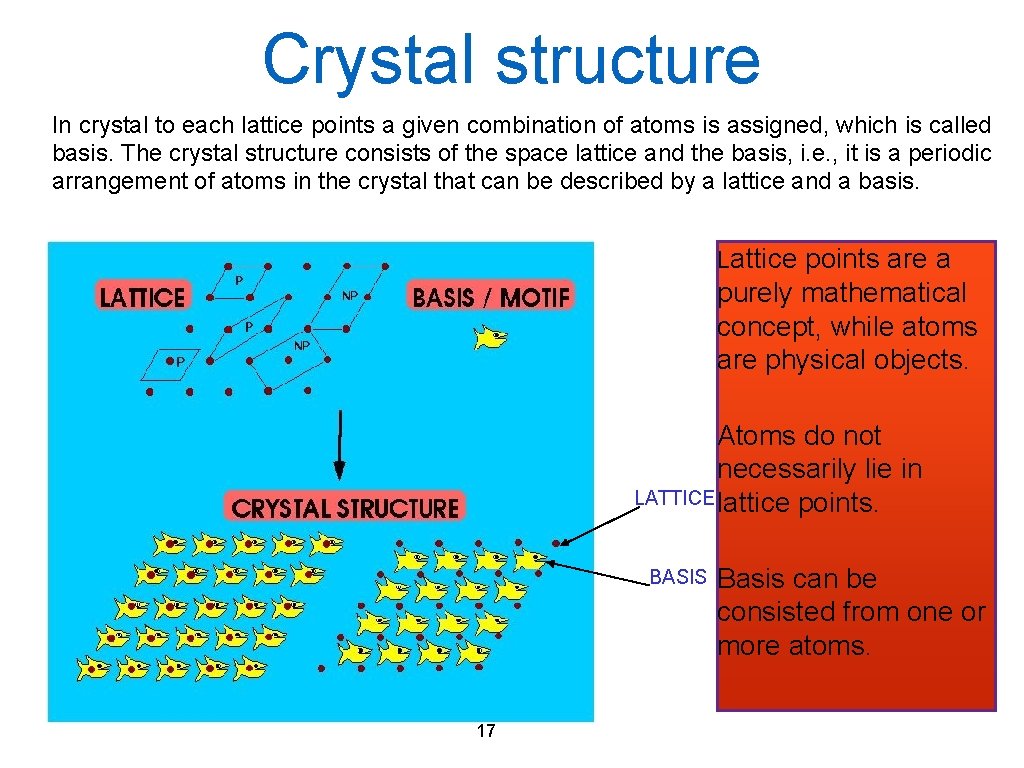
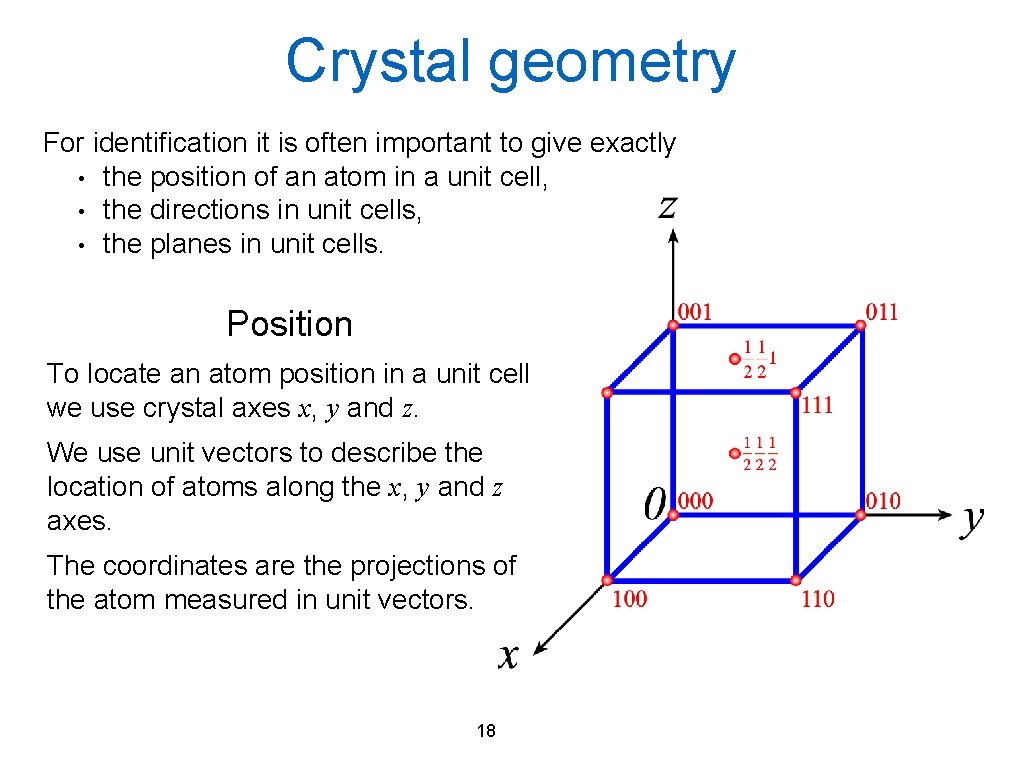
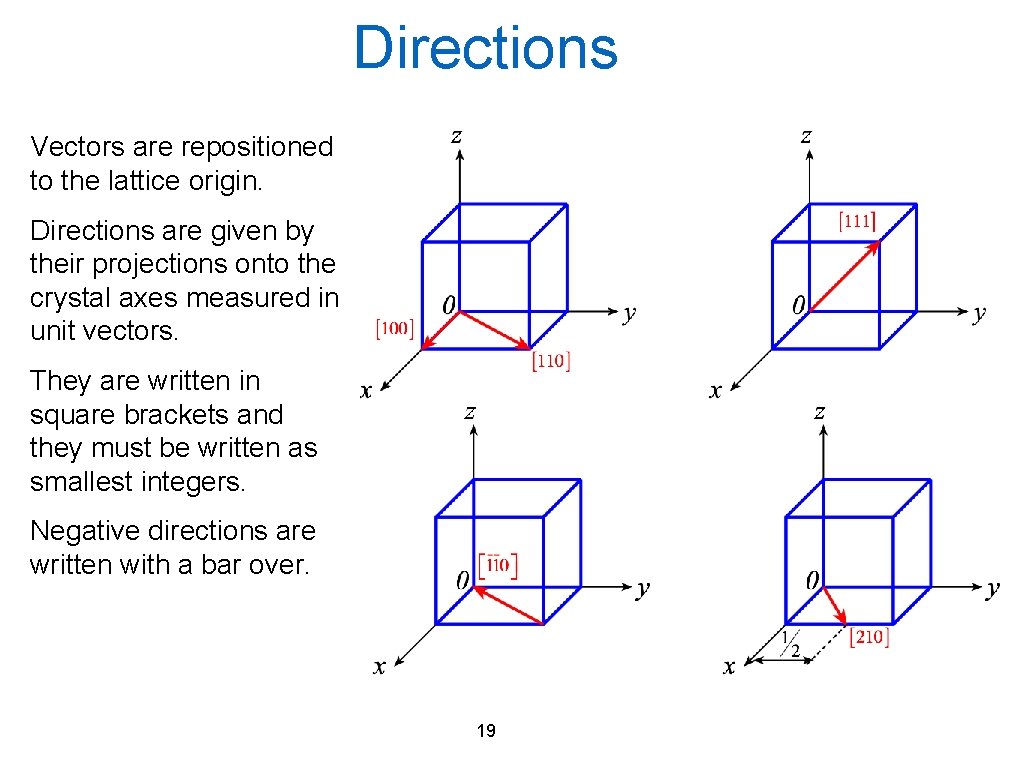
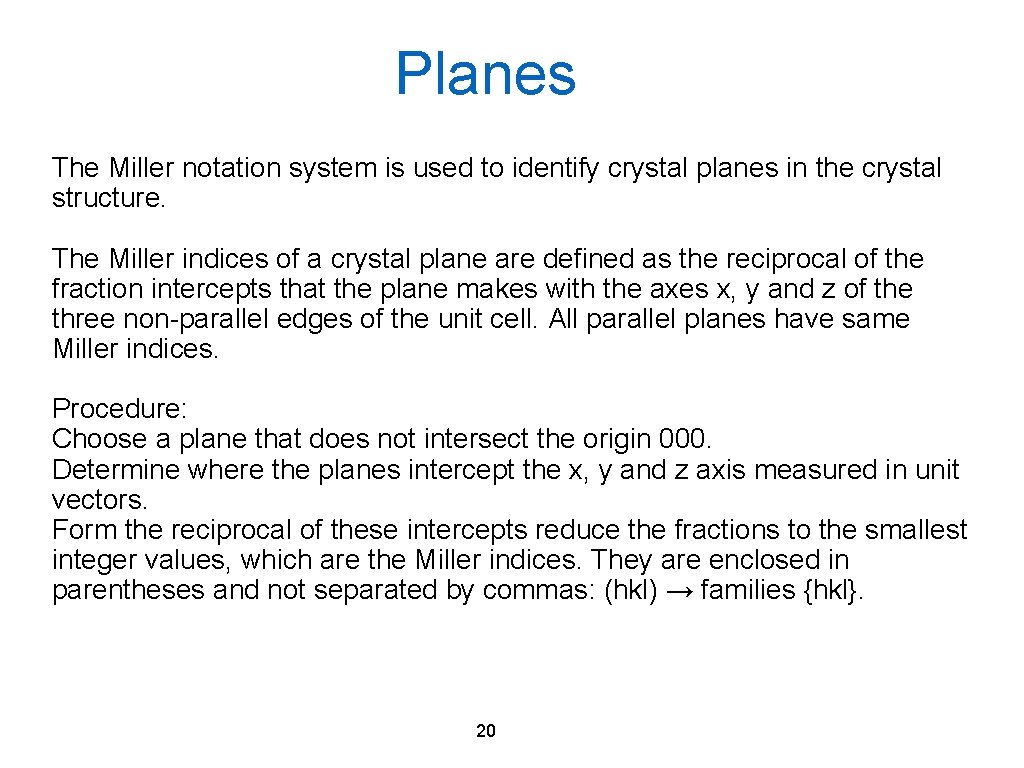
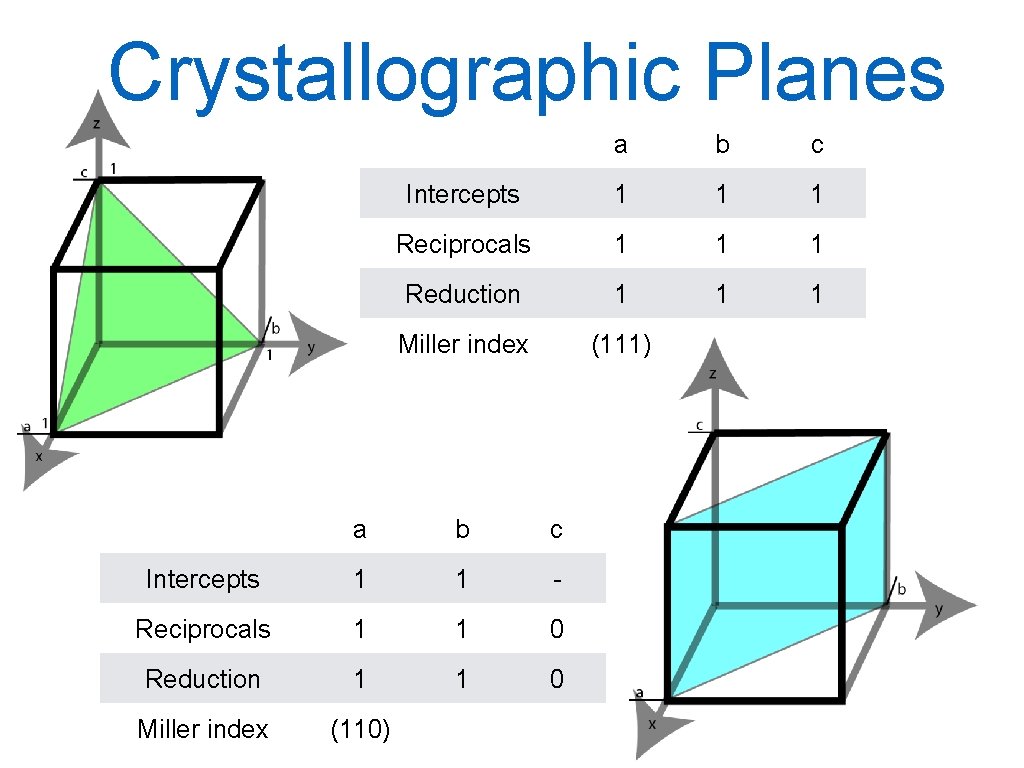
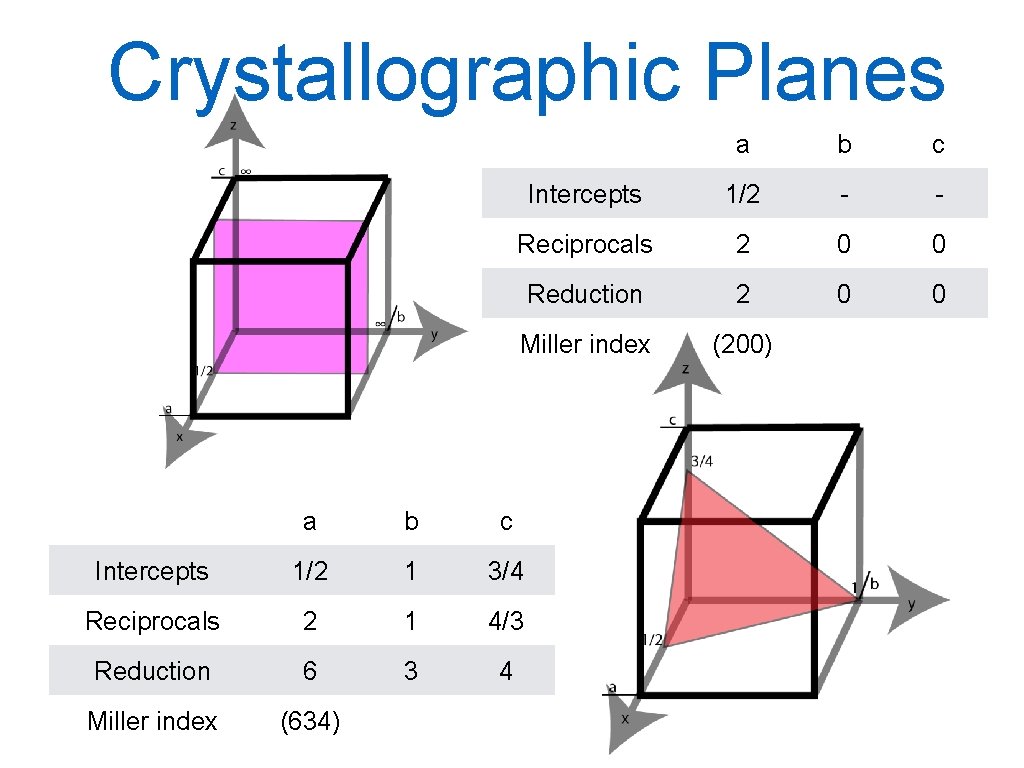
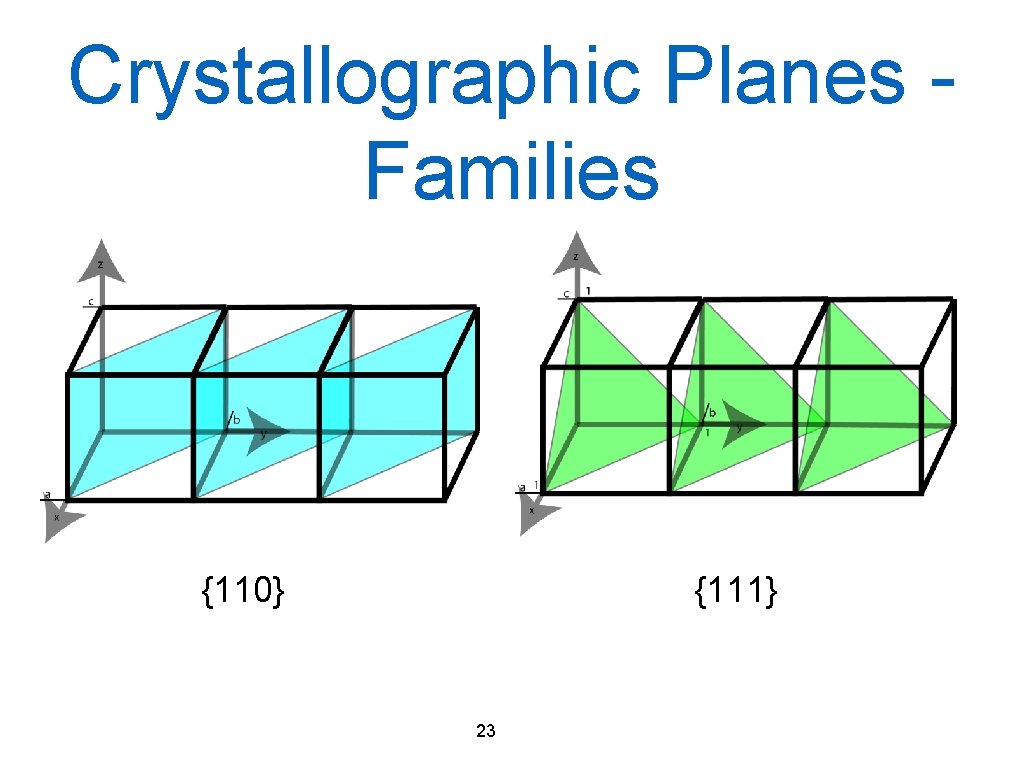
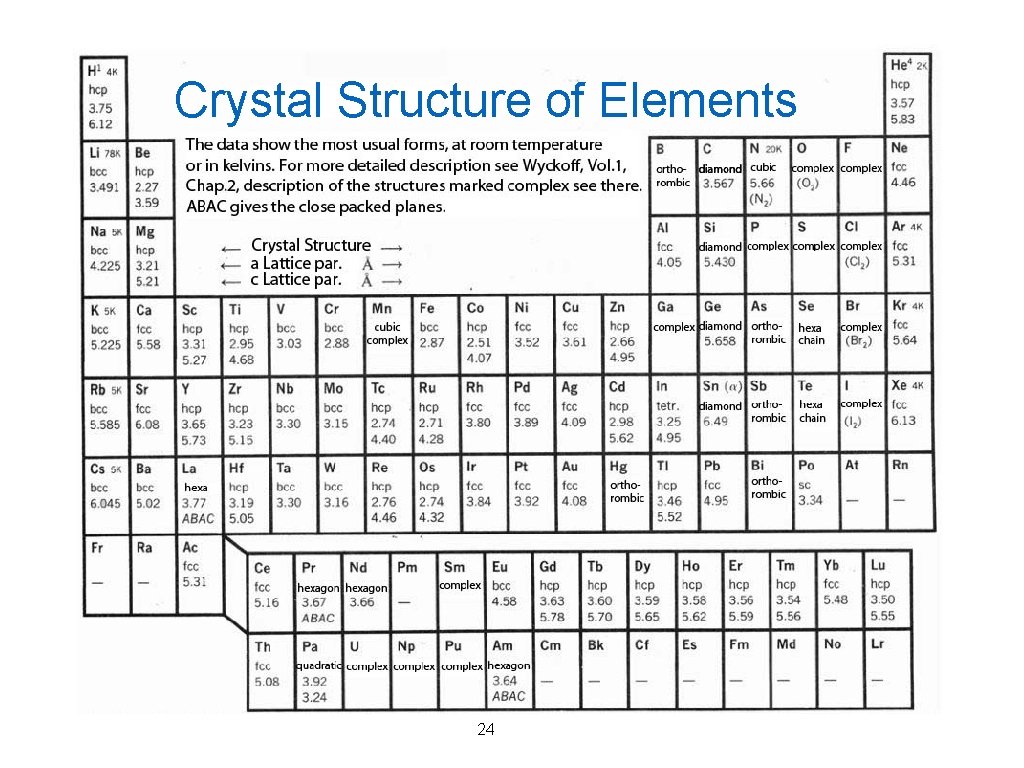
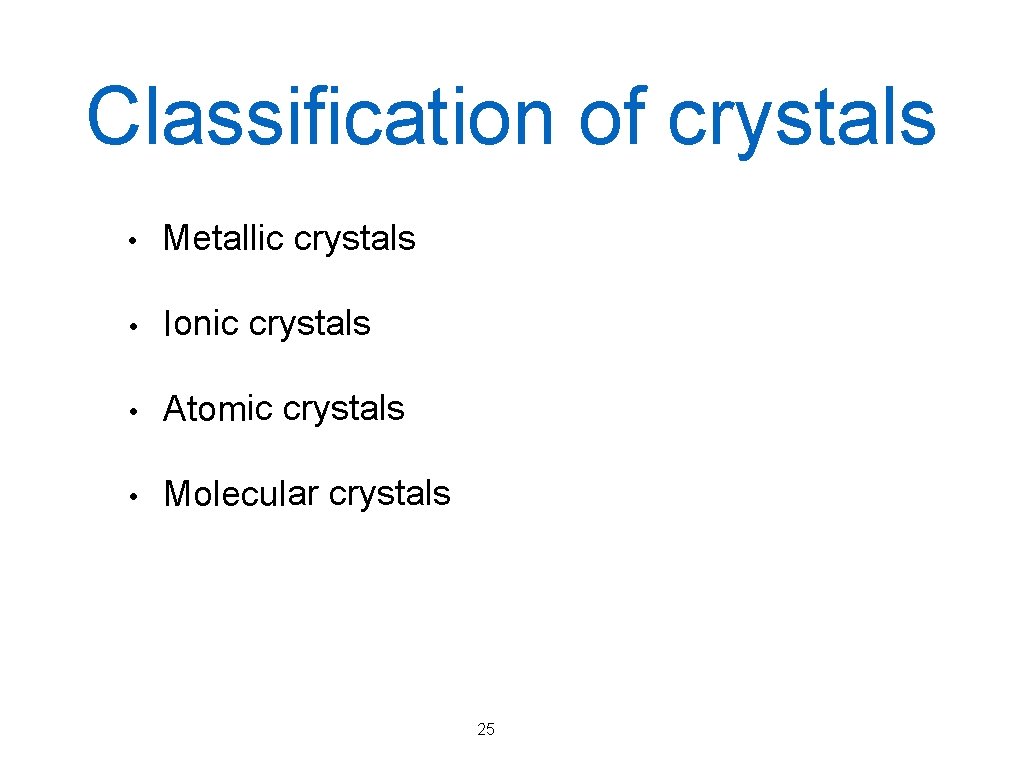
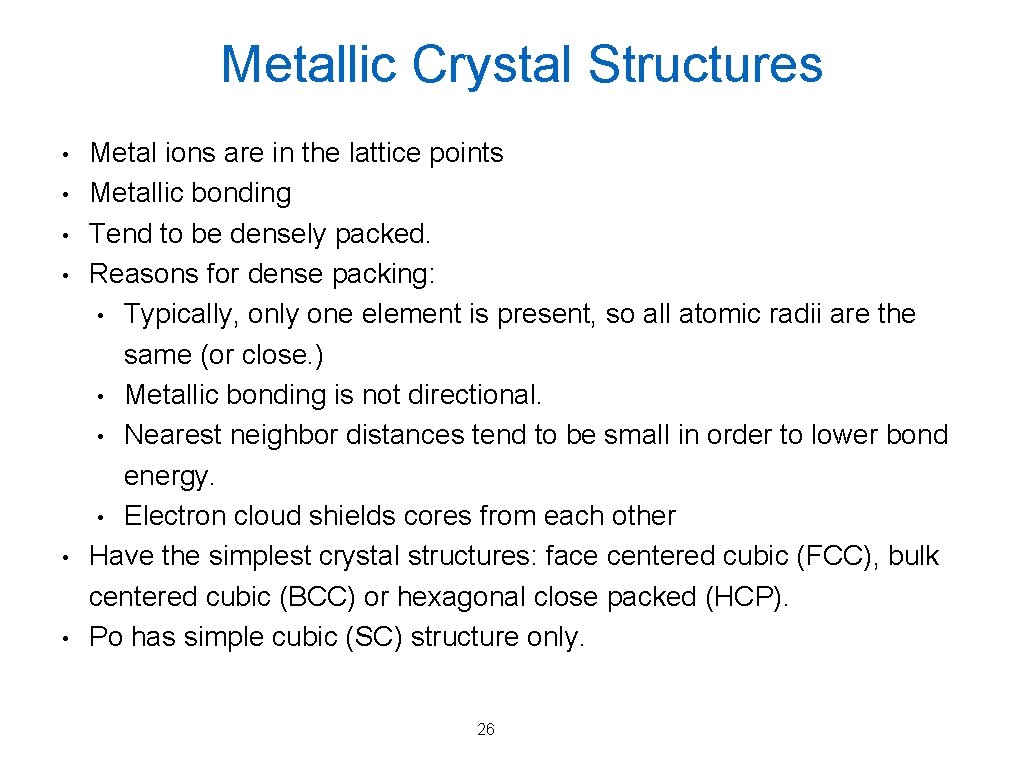
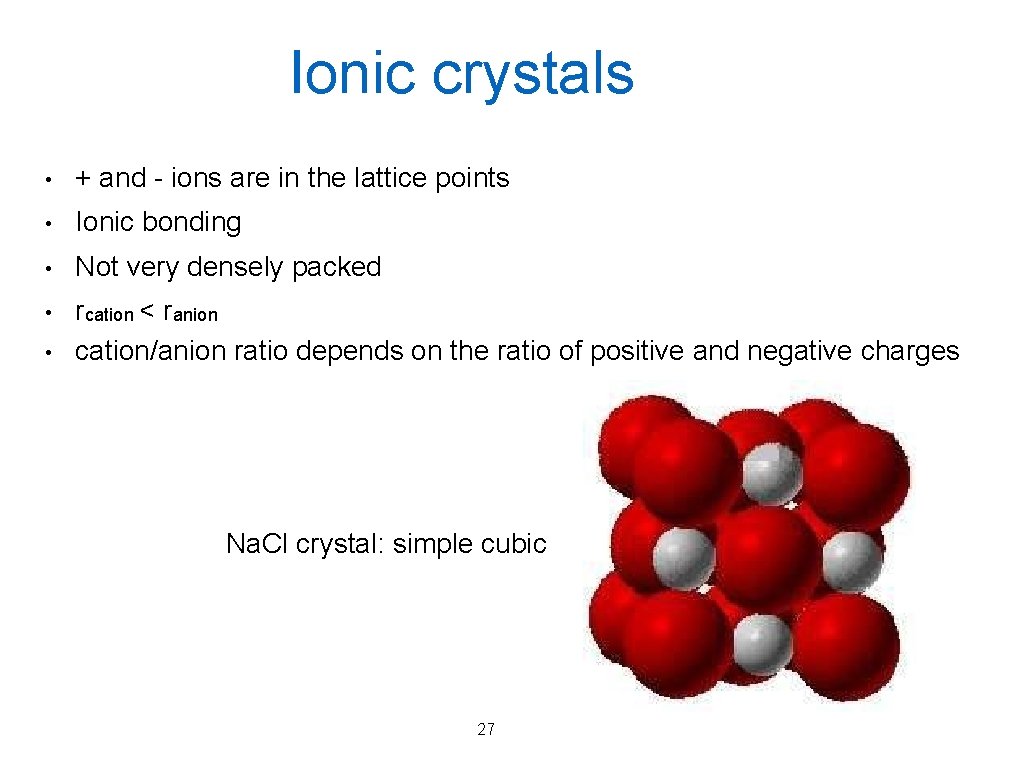
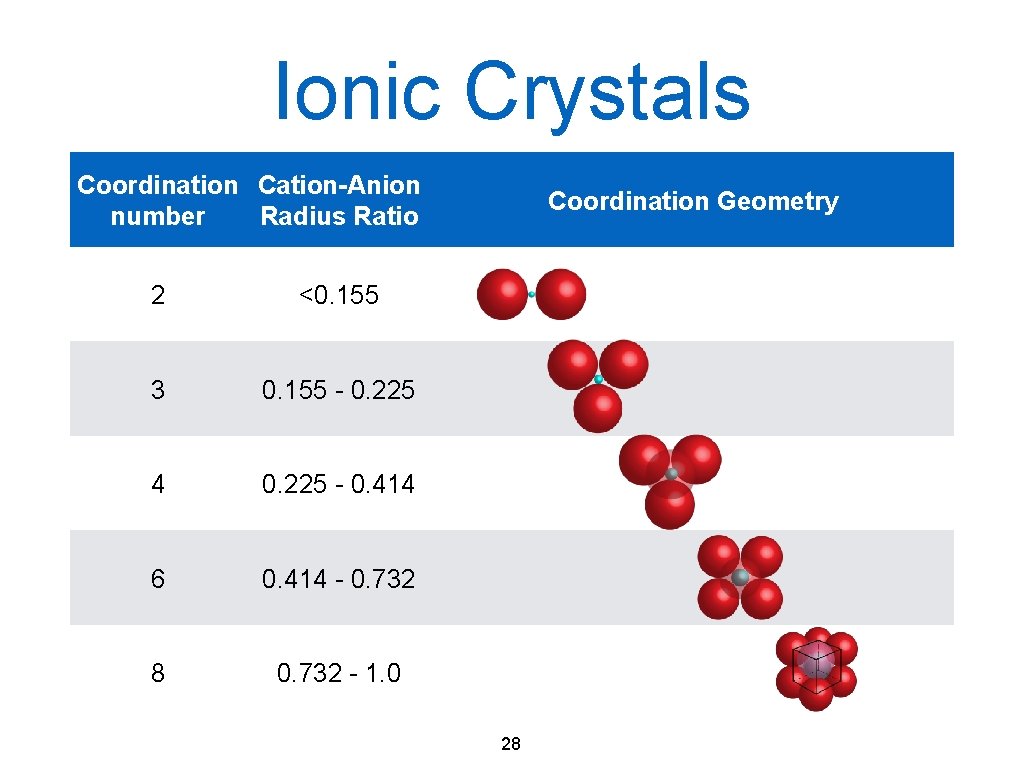
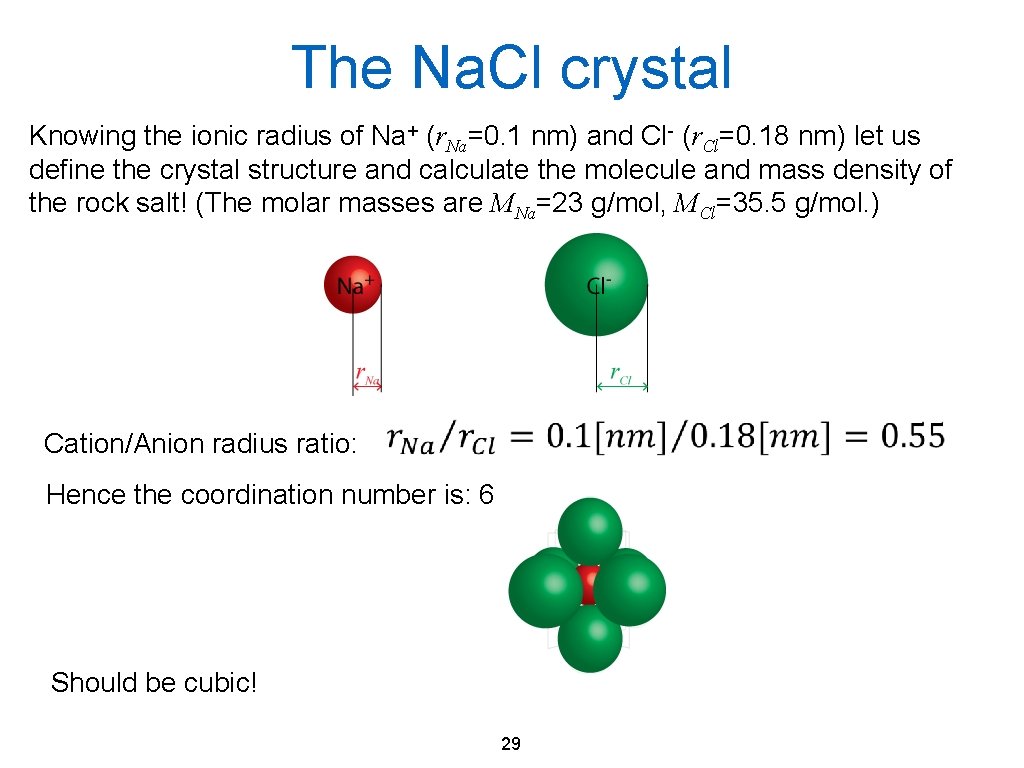
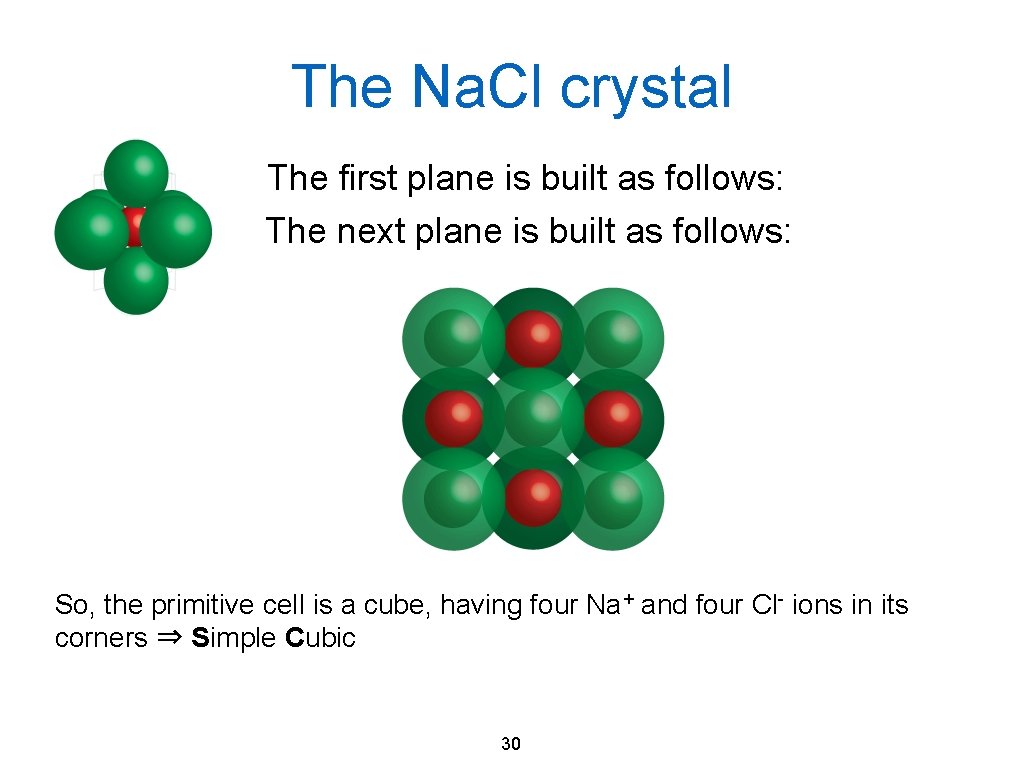
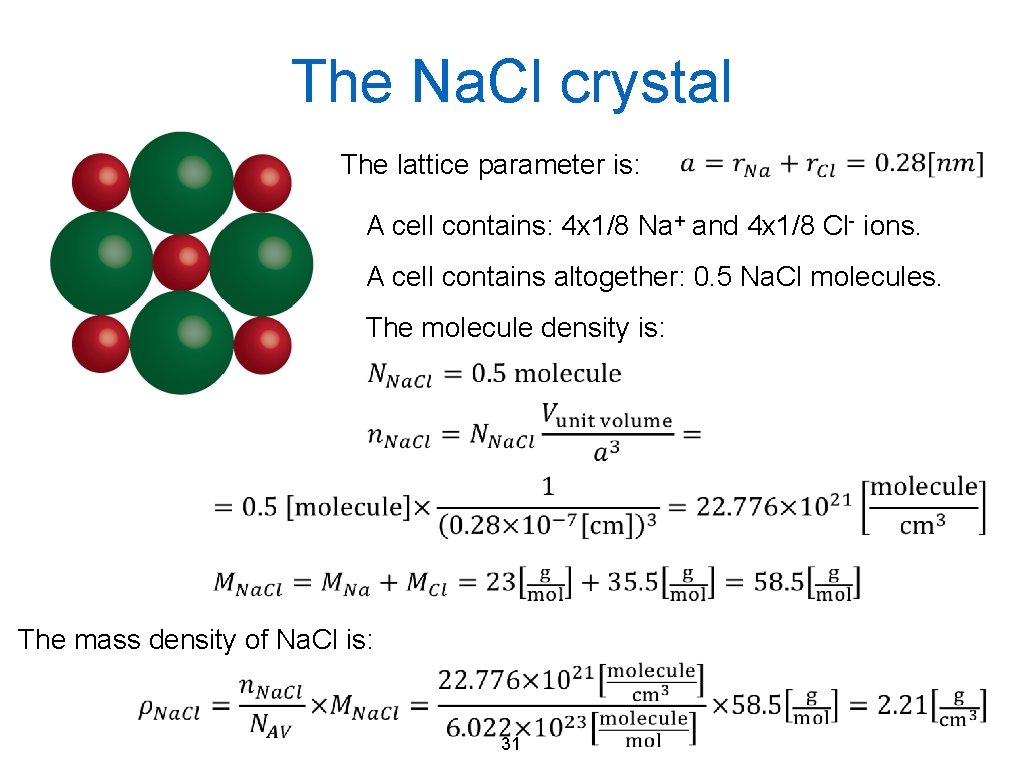
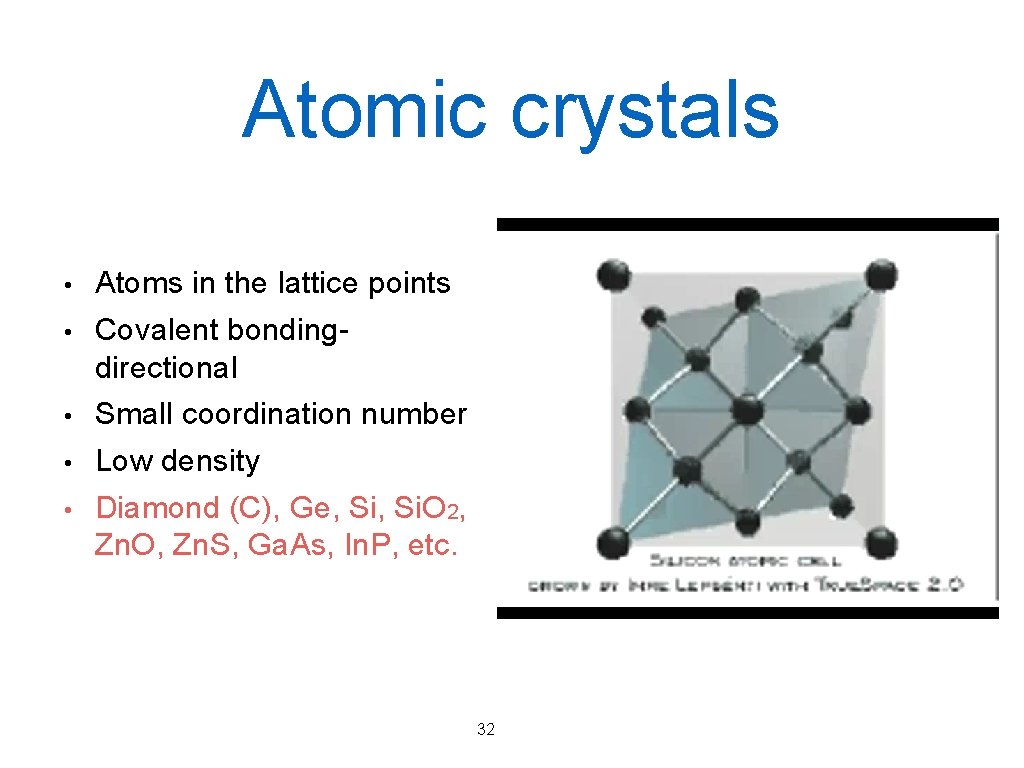
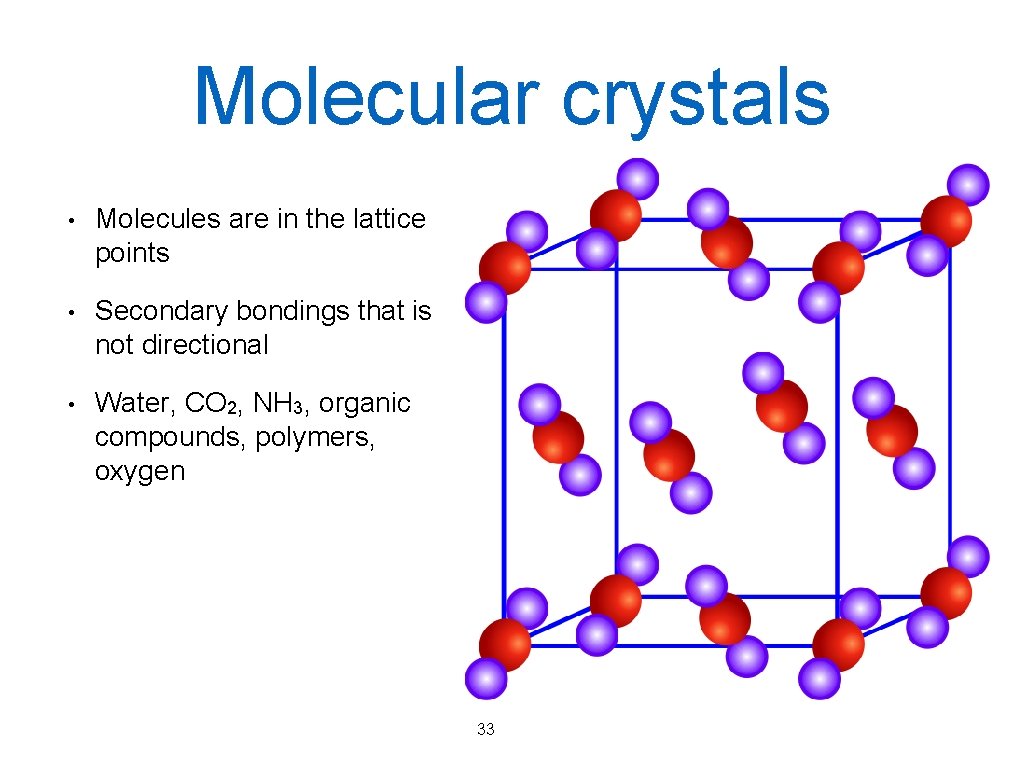
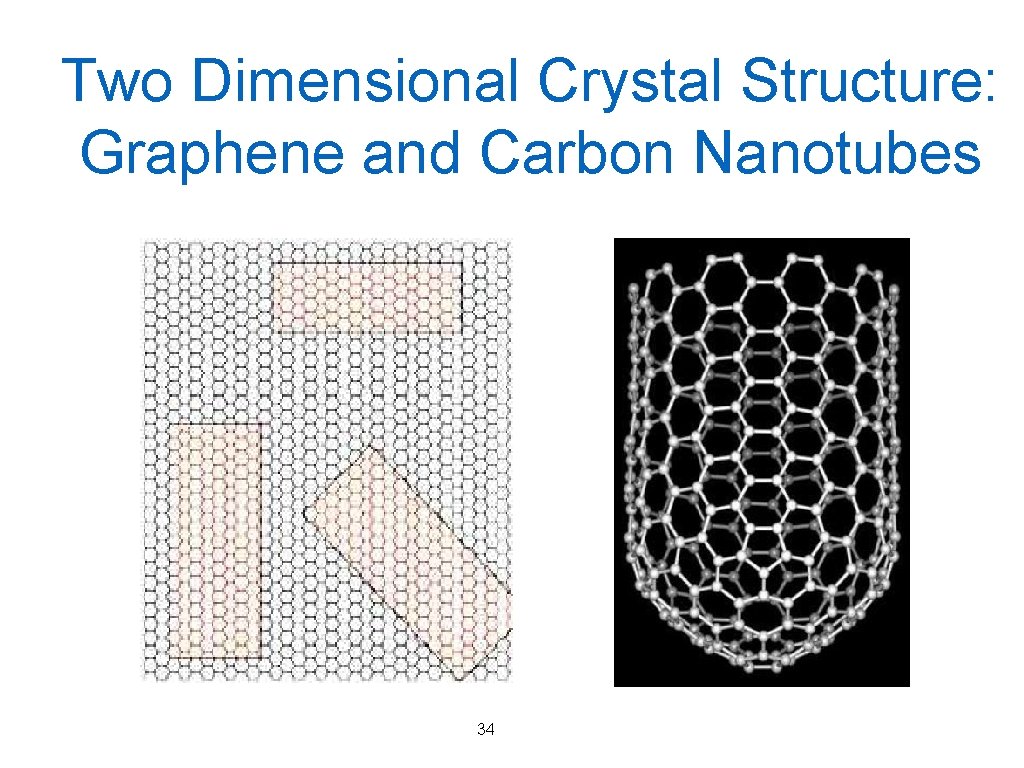
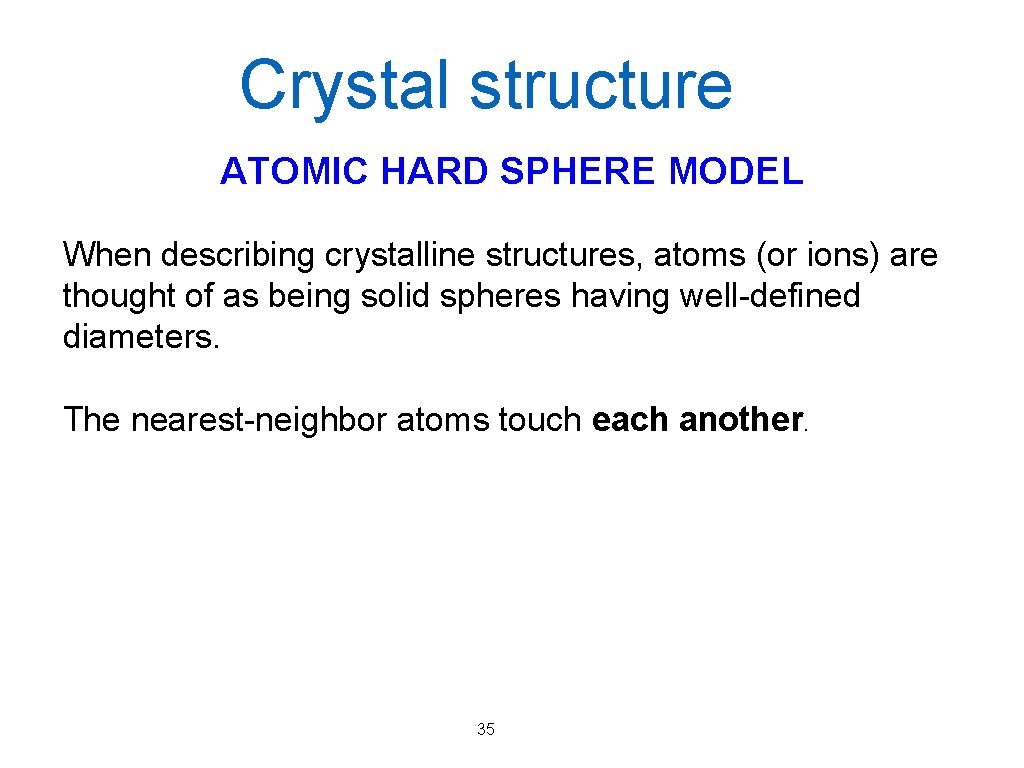
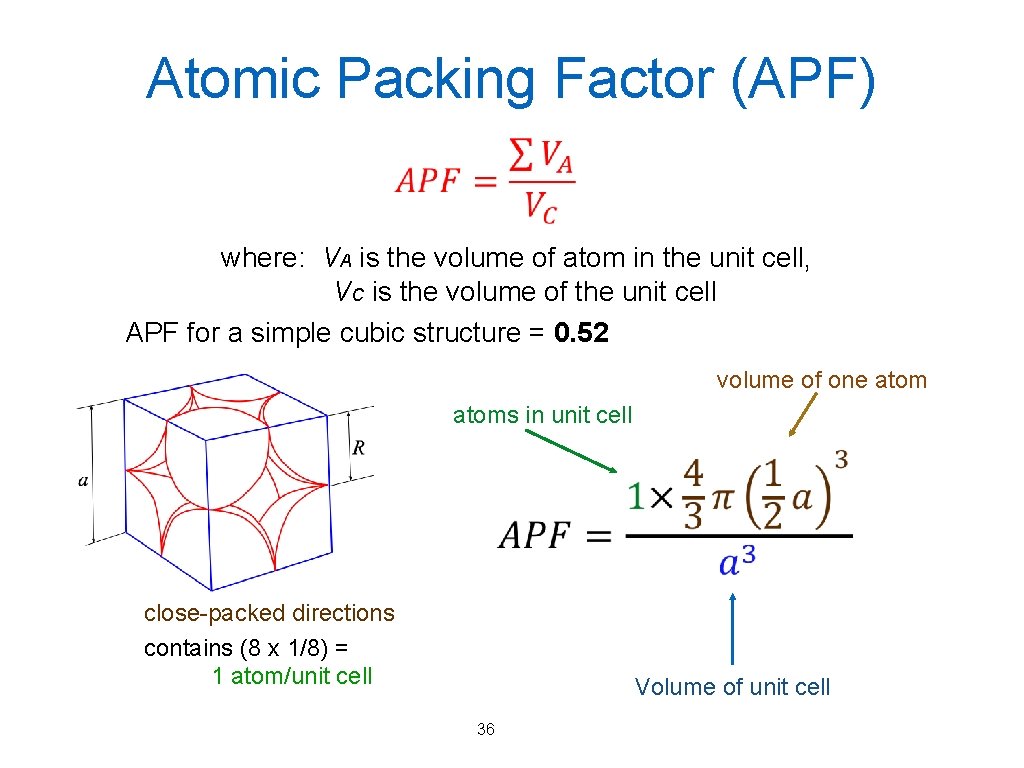
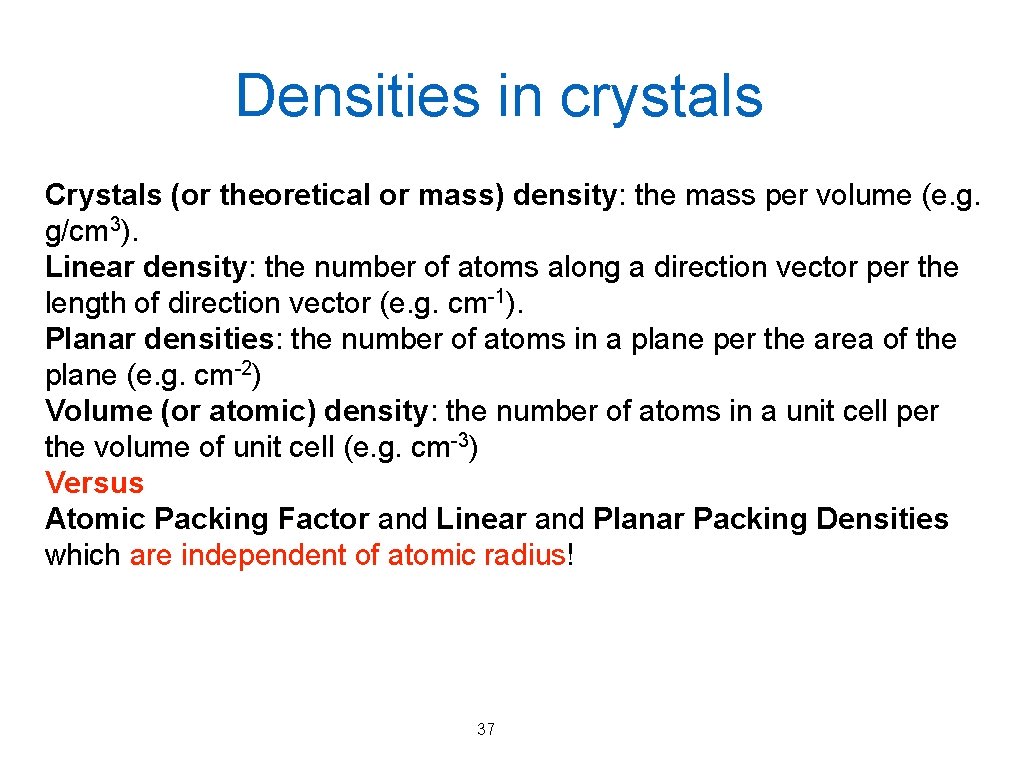
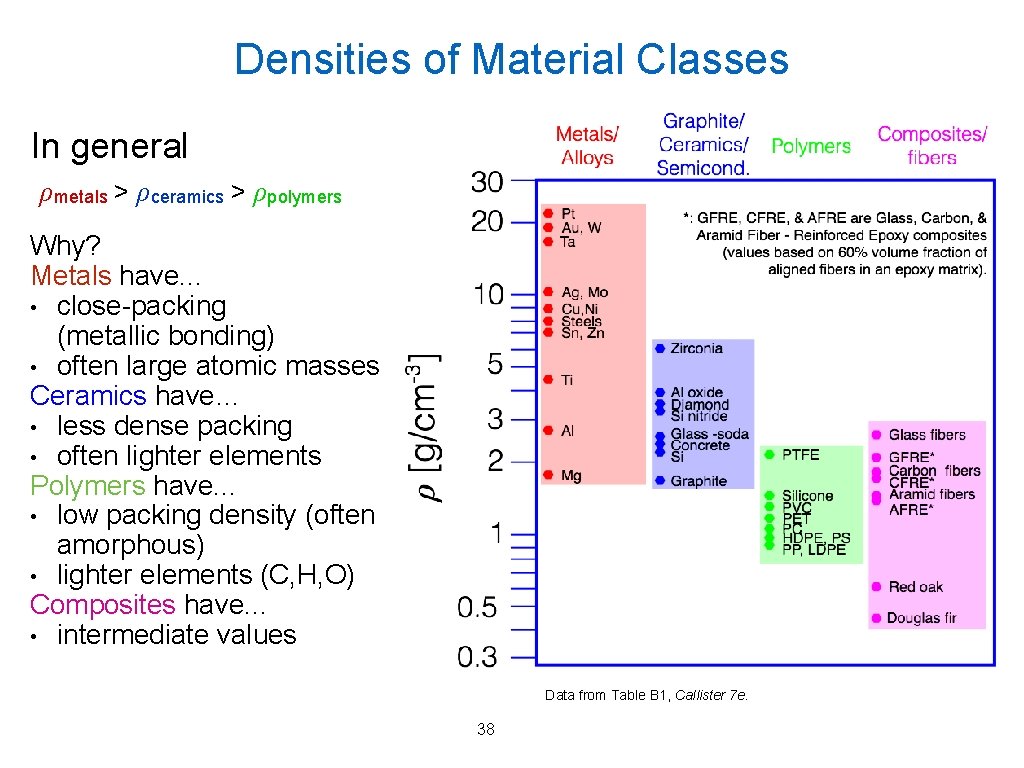
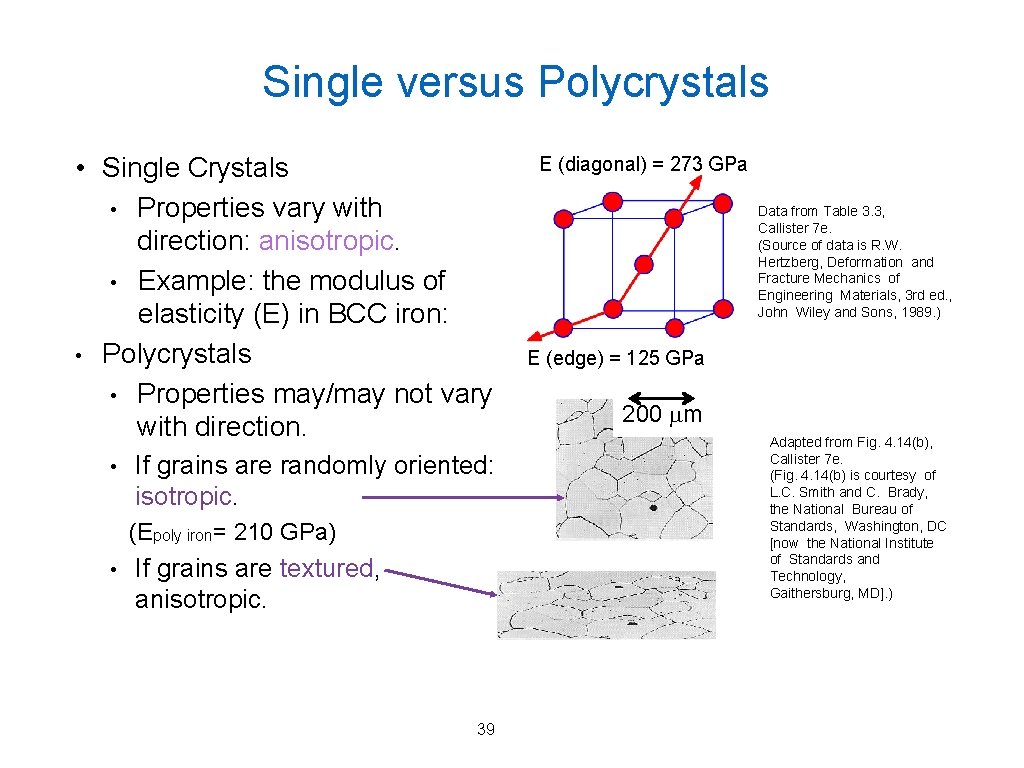
![Effects of Anisotropy: Modulus of Elasticity (GPa) Metal Structure [100] [111] Aluminum FCC 63. Effects of Anisotropy: Modulus of Elasticity (GPa) Metal Structure [100] [111] Aluminum FCC 63.](https://slidetodoc.com/presentation_image_h2/ee3cdeade56cc9d8b89c2d84a5f2ebb2/image-40.jpg)
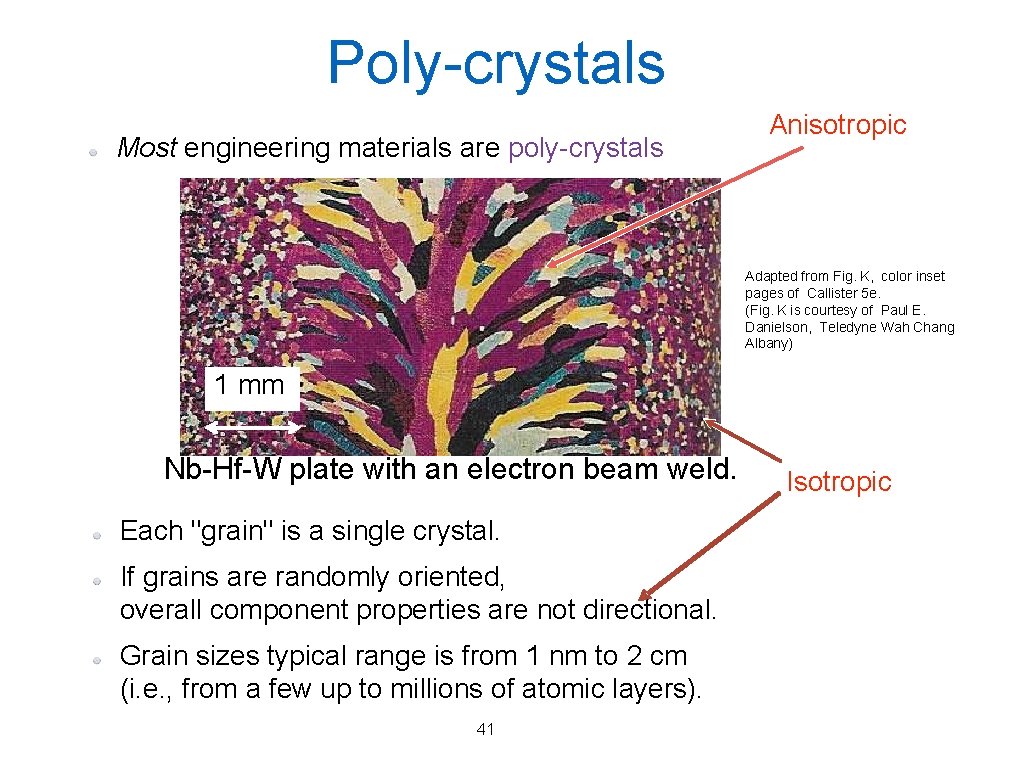
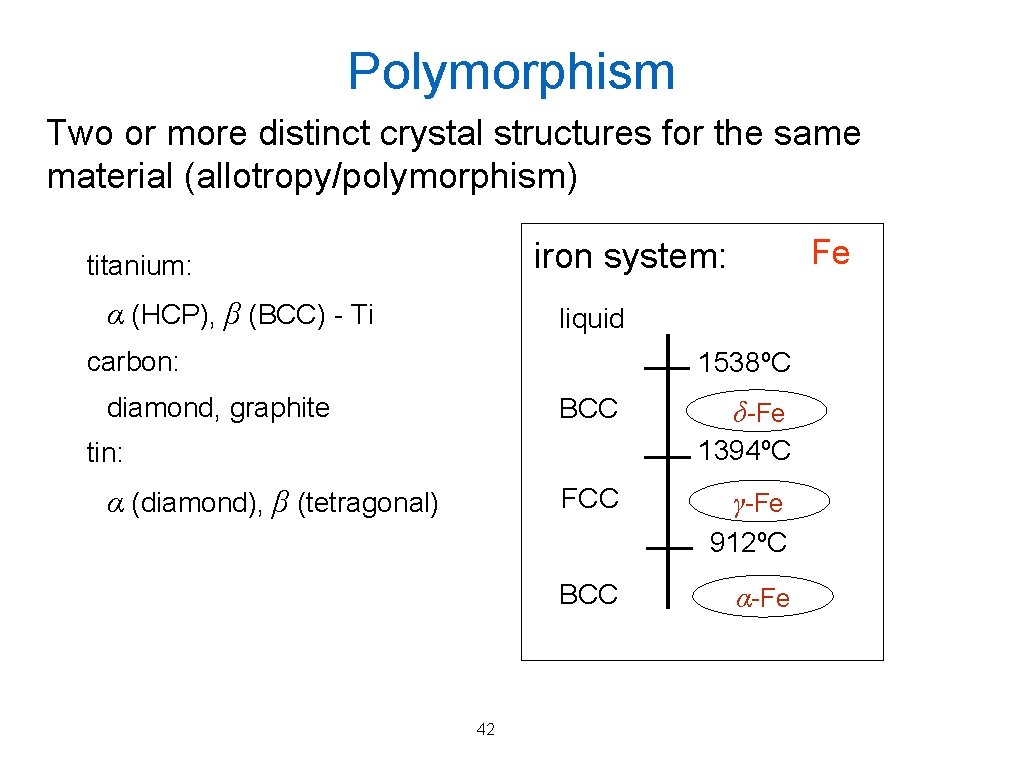
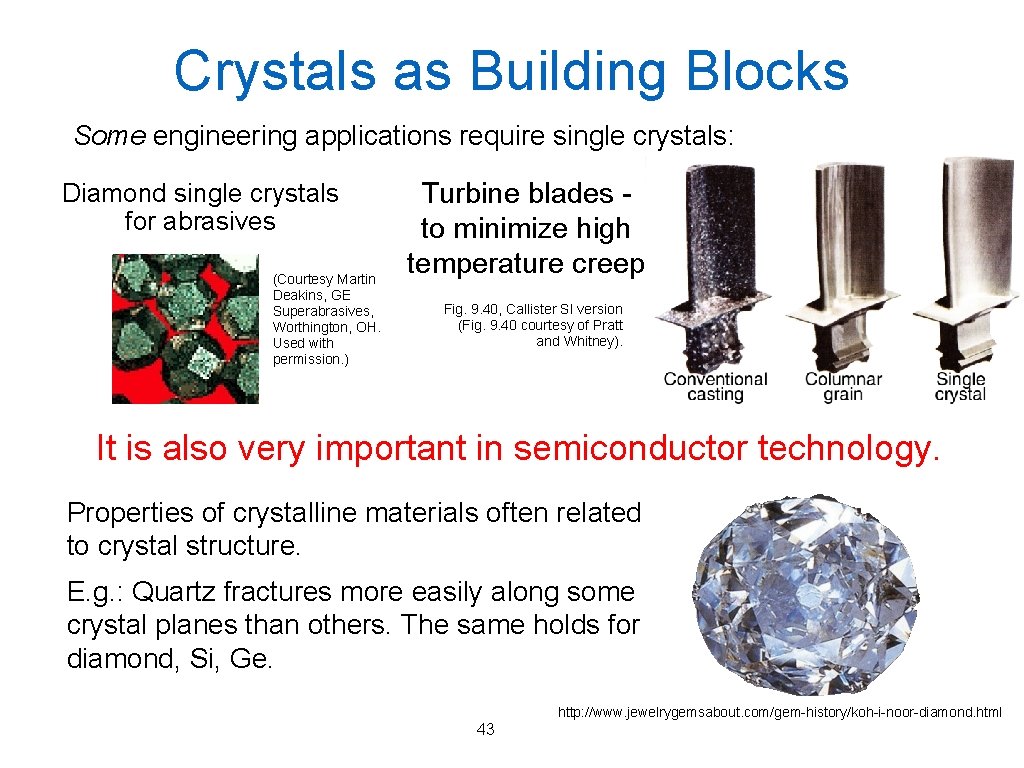
- Slides: 43
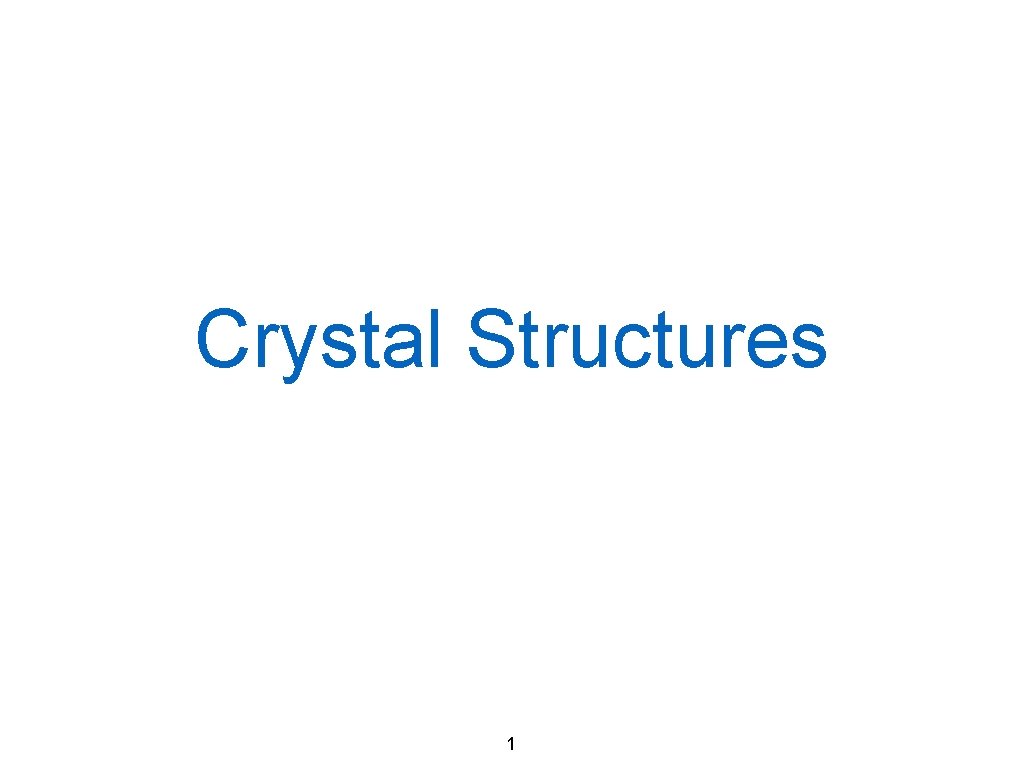
Crystal Structures 1
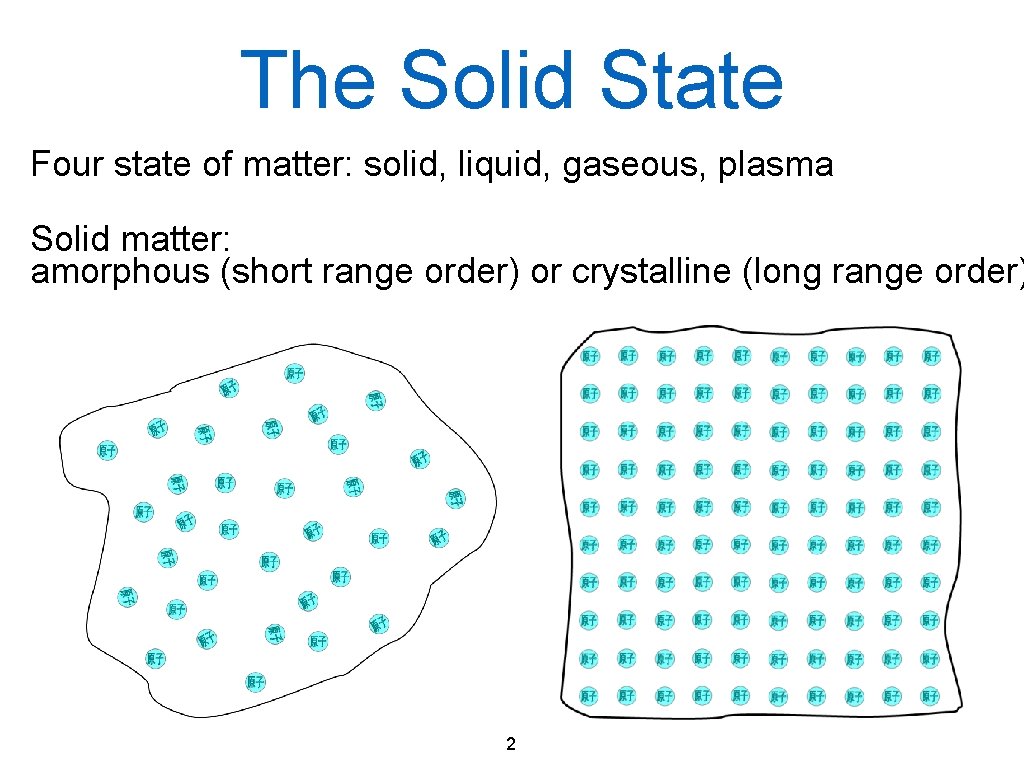
The Solid State Four state of matter: solid, liquid, gaseous, plasma Solid matter: amorphous (short range order) or crystalline (long range order) 2
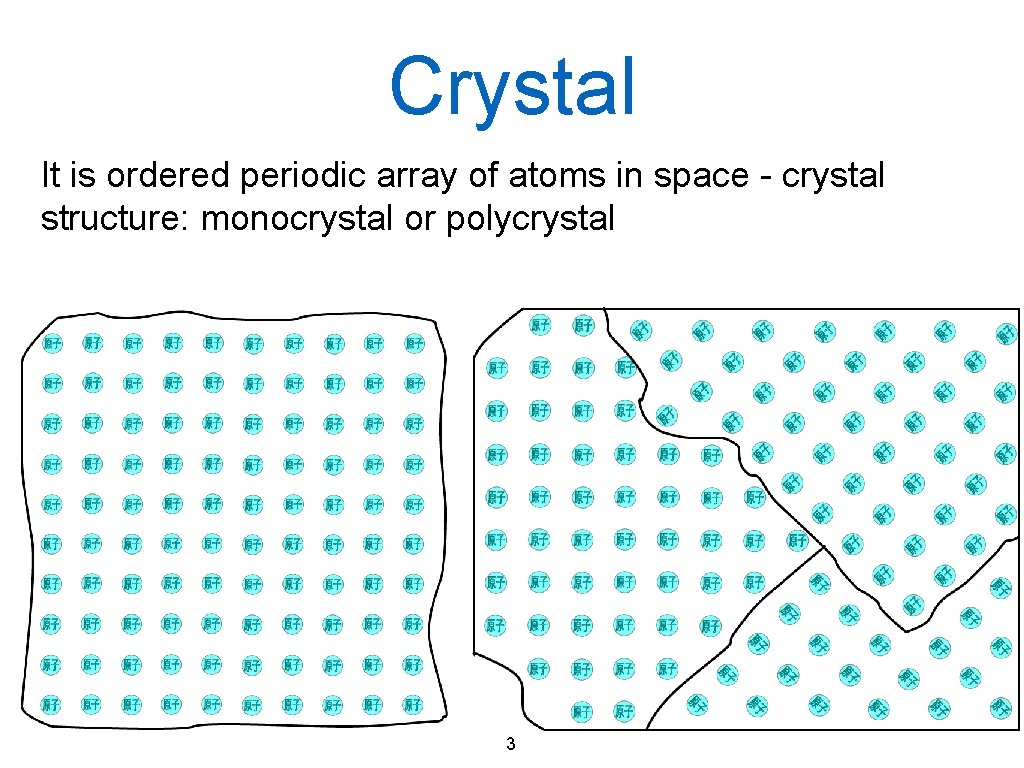
Crystal It is ordered periodic array of atoms in space - crystal structure: monocrystal or polycrystal 3
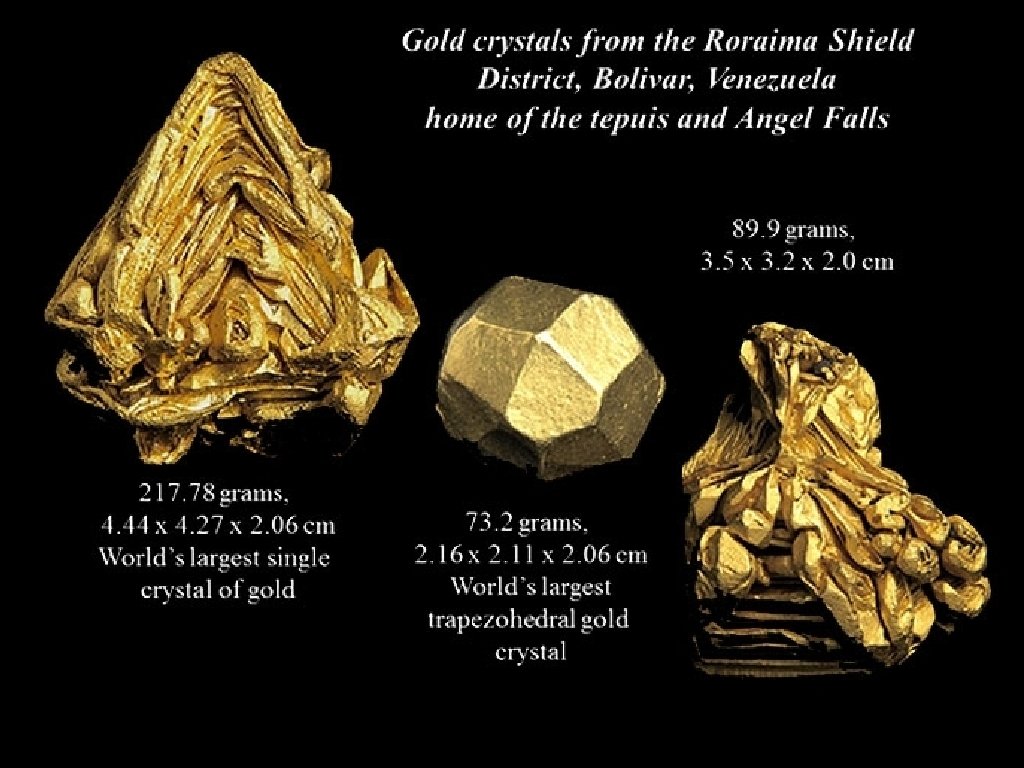
4
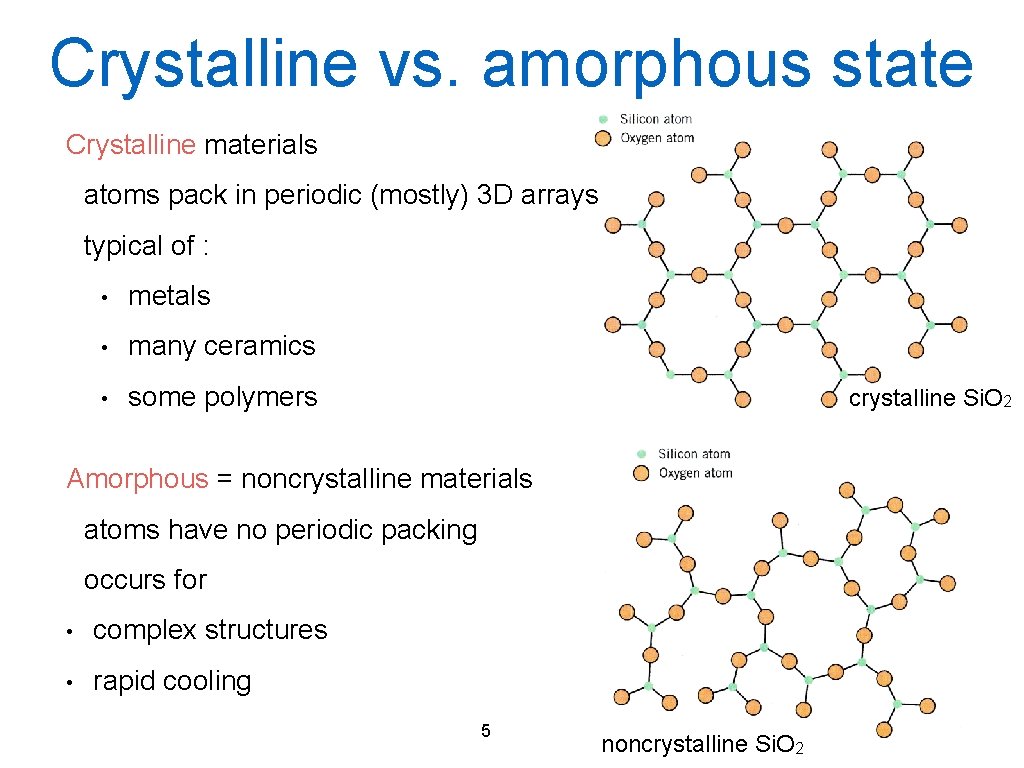
Crystalline vs. amorphous state Crystalline materials atoms pack in periodic (mostly) 3 D arrays typical of : • metals • many ceramics • some polymers crystalline Si. O 2 Amorphous = noncrystalline materials atoms have no periodic packing occurs for • complex structures • rapid cooling 5 noncrystalline Si. O 2
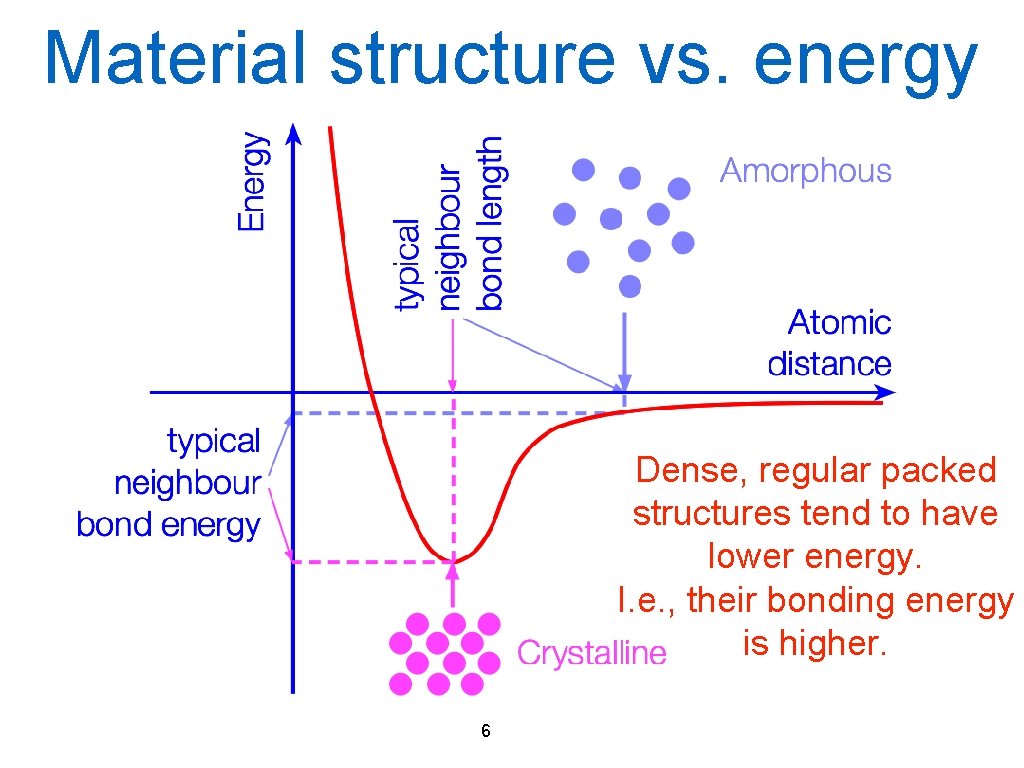
Material structure vs. energy Dense, regular packed structures tend to have lower energy. I. e. , their bonding energy is higher. 6
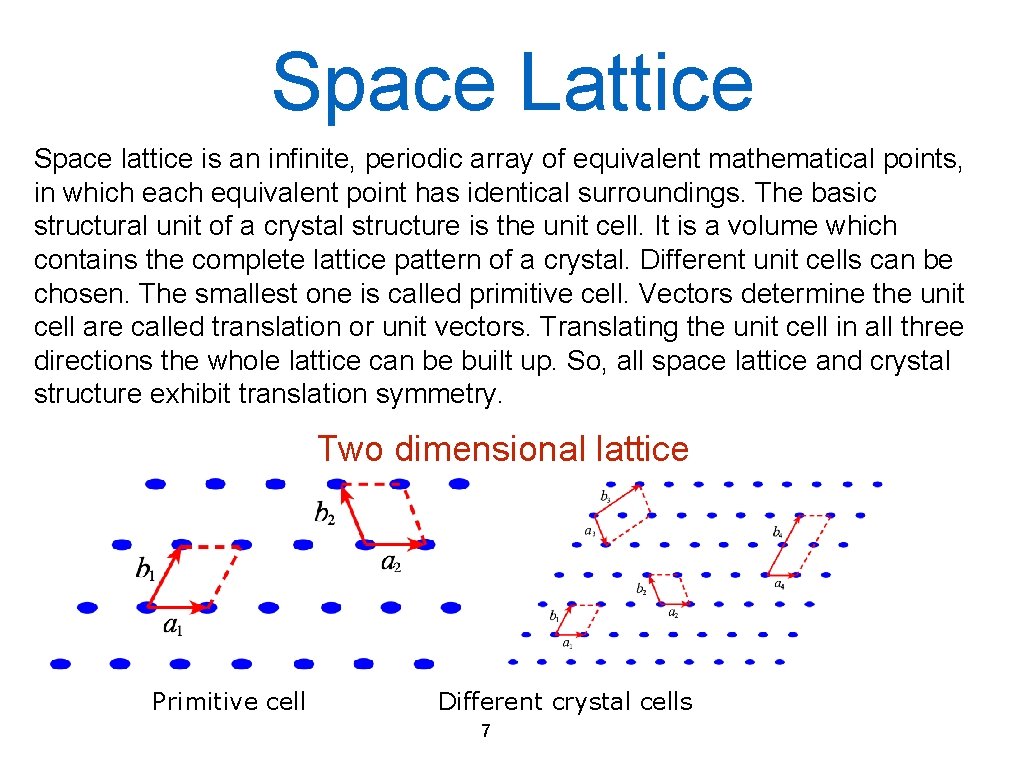
Space Lattice Space lattice is an infinite, periodic array of equivalent mathematical points, in which each equivalent point has identical surroundings. The basic structural unit of a crystal structure is the unit cell. It is a volume which contains the complete lattice pattern of a crystal. Different unit cells can be chosen. The smallest one is called primitive cell. Vectors determine the unit cell are called translation or unit vectors. Translating the unit cell in all three directions the whole lattice can be built up. So, all space lattice and crystal structure exhibit translation symmetry. Two dimensional lattice Primitive cell Different crystal cells 7
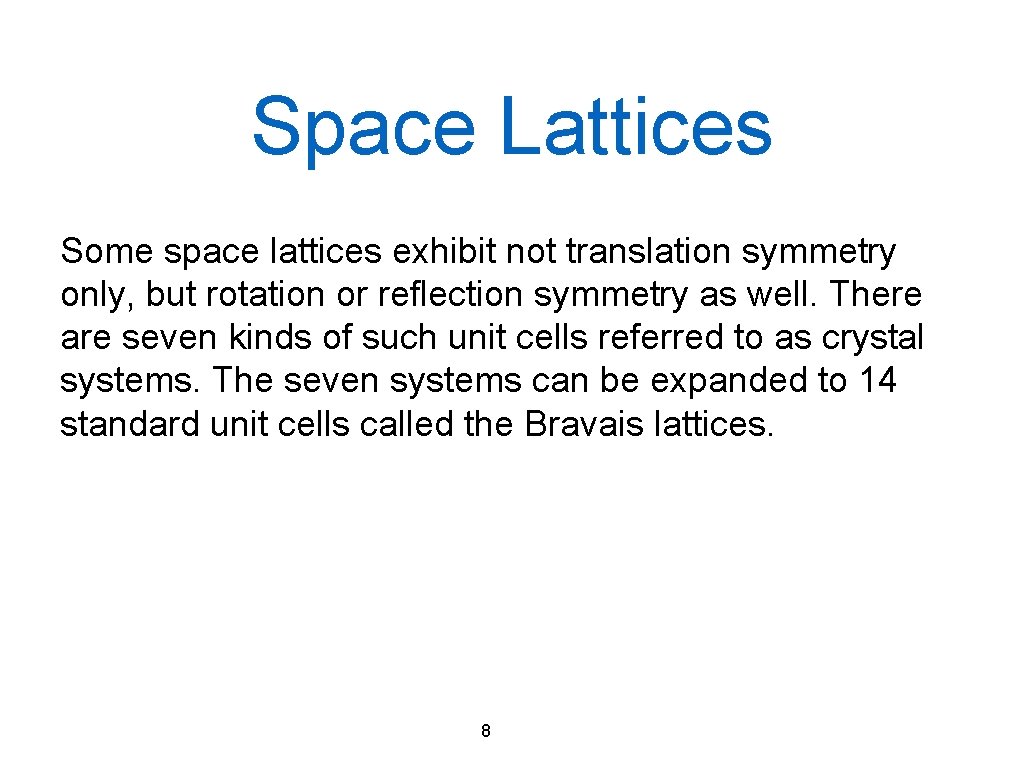
Space Lattices Some space lattices exhibit not translation symmetry only, but rotation or reflection symmetry as well. There are seven kinds of such unit cells referred to as crystal systems. The seven systems can be expanded to 14 standard unit cells called the Bravais lattices. 8
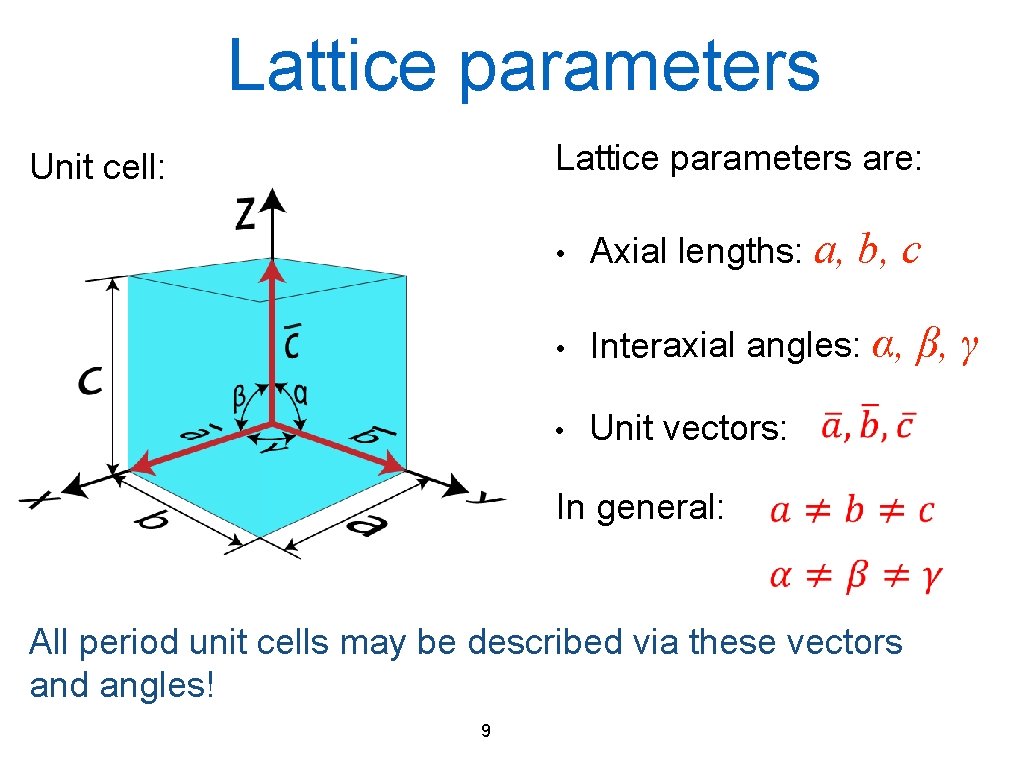
Lattice parameters are: Unit cell: b, c • Axial lengths: a, • Interaxial angles: α, • Unit vectors: In general: All period unit cells may be described via these vectors and angles! 9 β, γ
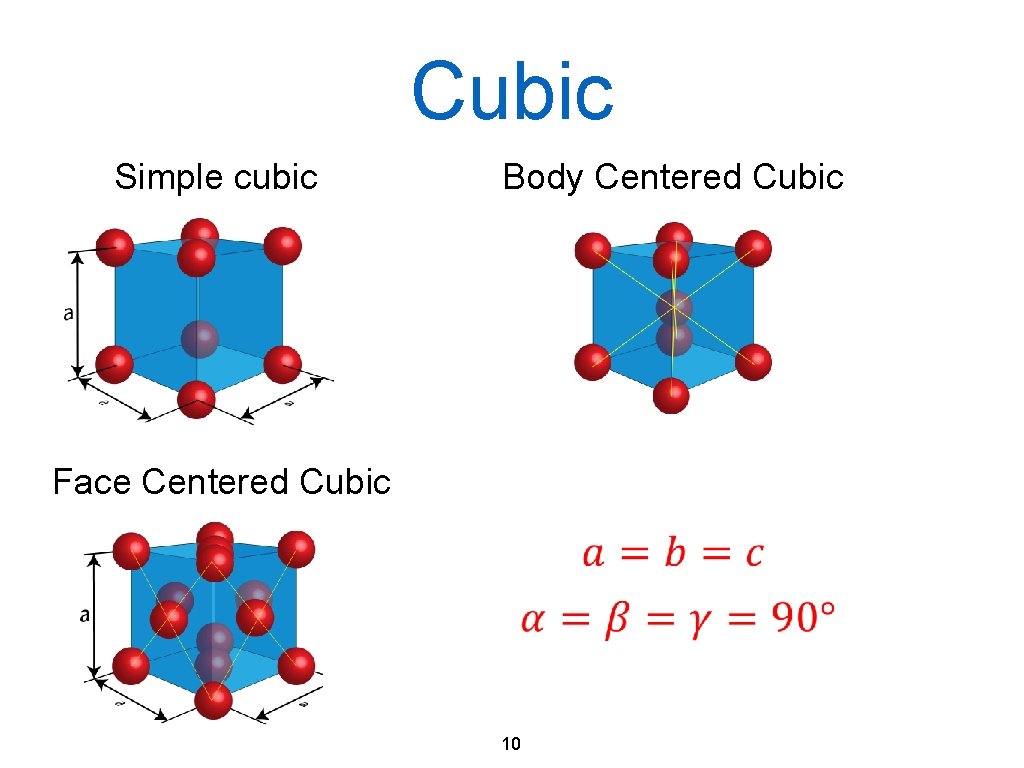
Cubic Simple cubic Body Centered Cubic Face Centered Cubic 10
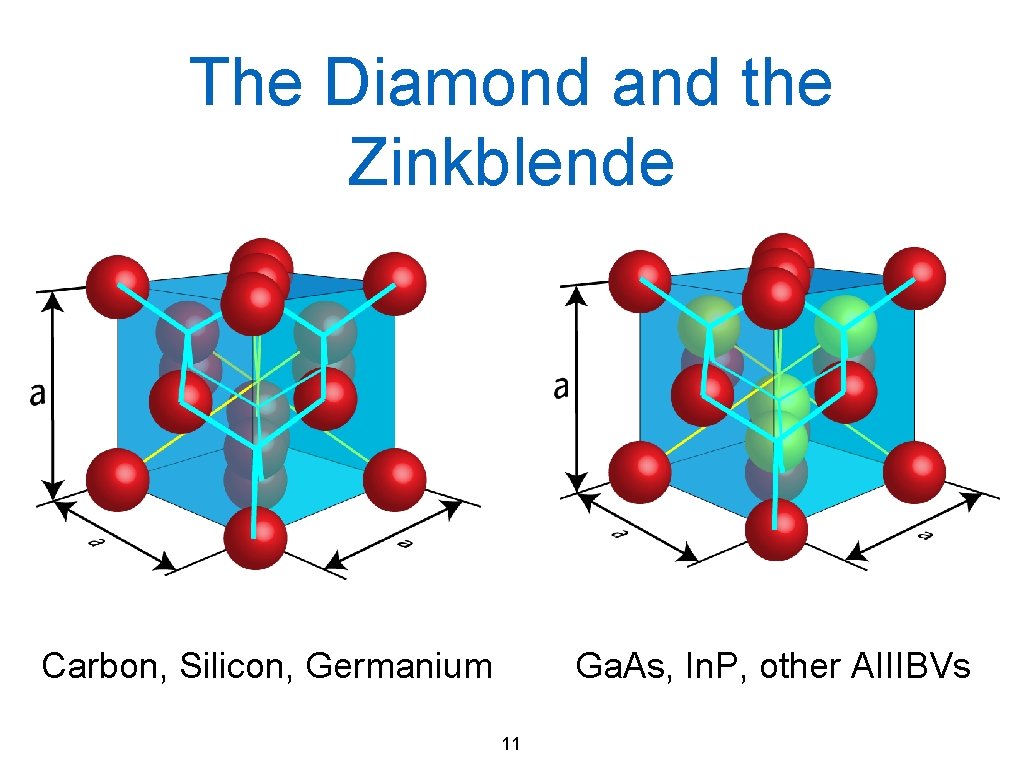
The Diamond and the Zinkblende Carbon, Silicon, Germanium Ga. As, In. P, other AIIIBVs 11
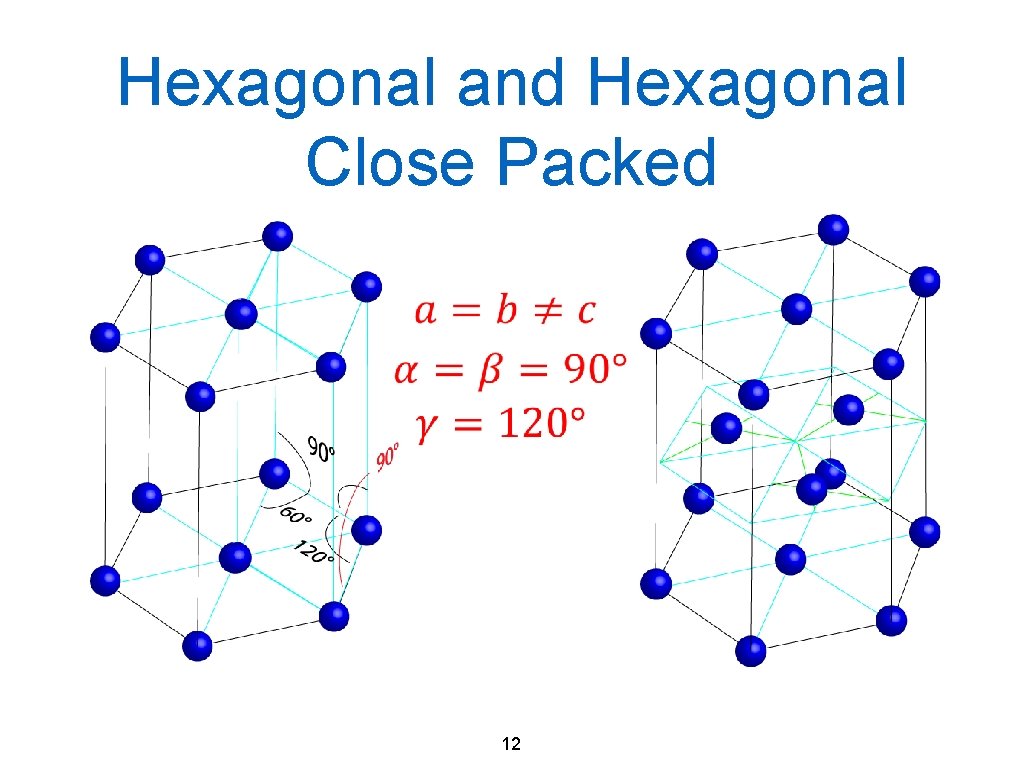
Hexagonal and Hexagonal Close Packed 12
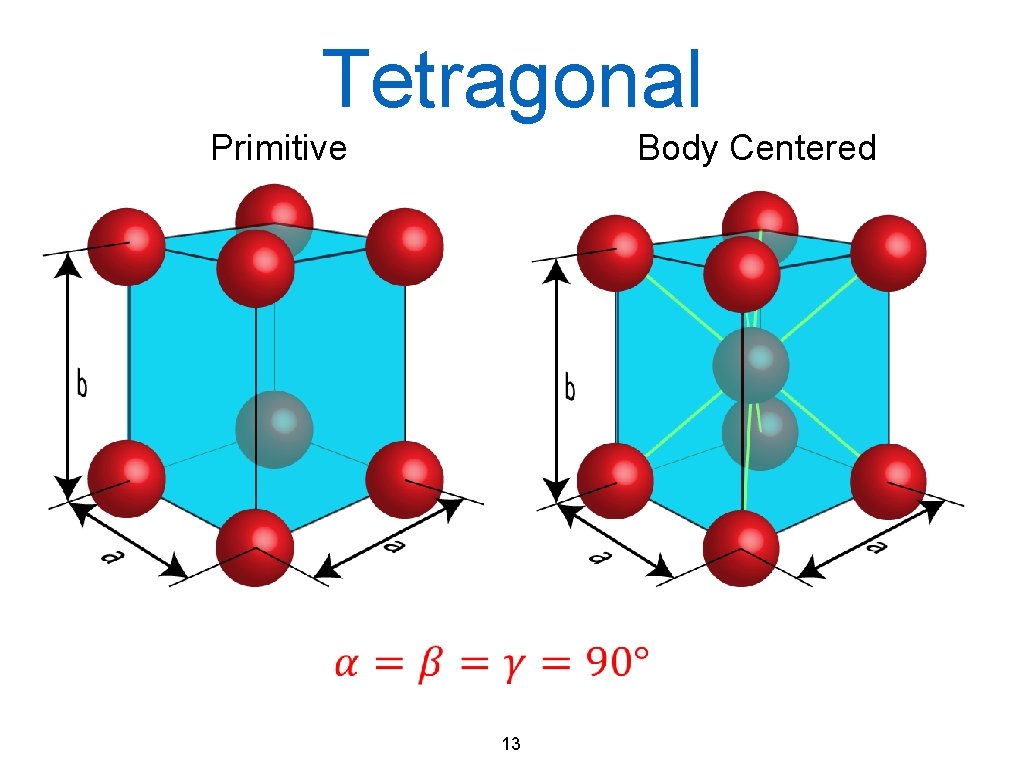
Tetragonal Primitive Body Centered 13
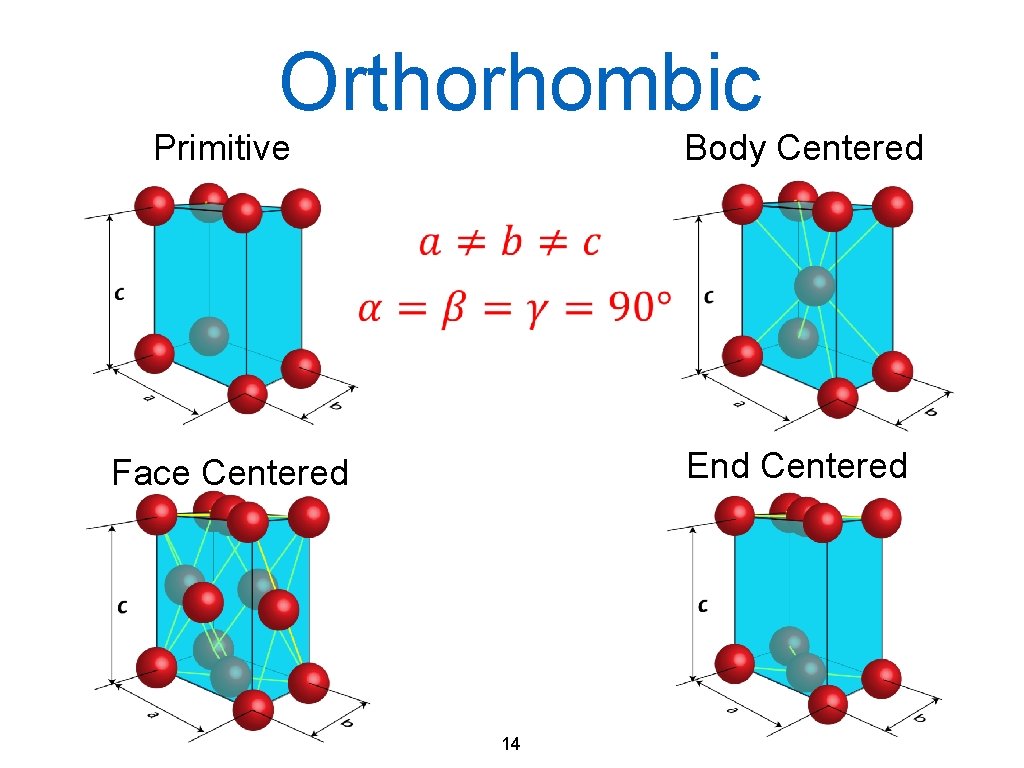
Orthorhombic Primitive Body Centered Face Centered End Centered 14
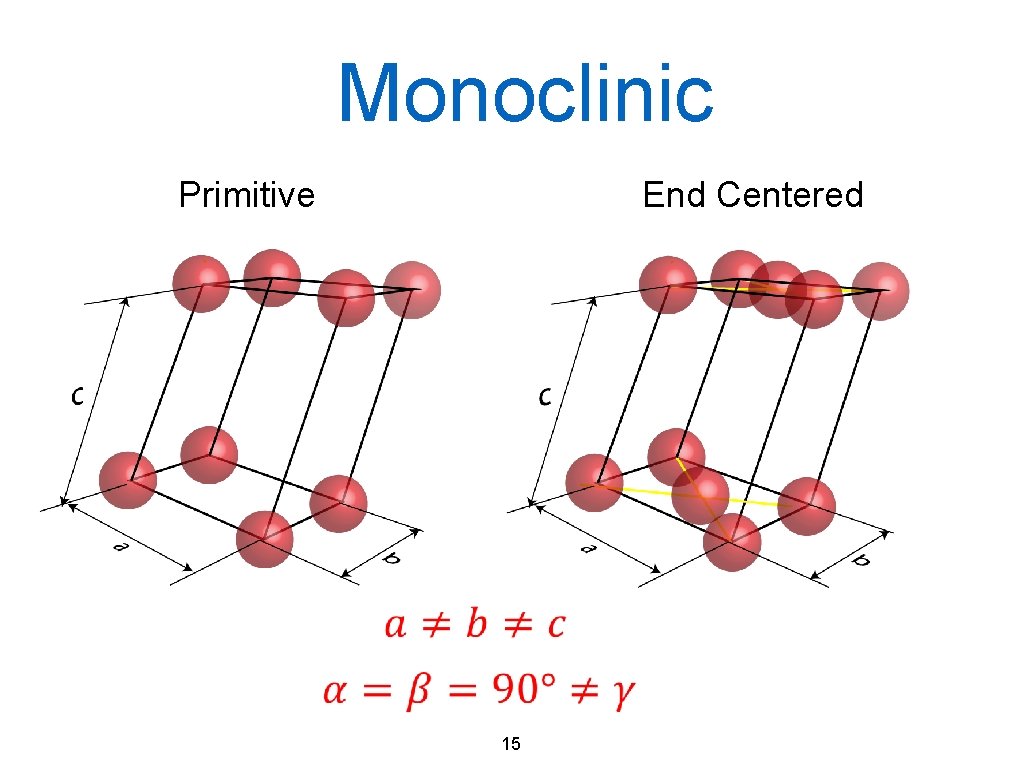
Monoclinic Primitive End Centered 15
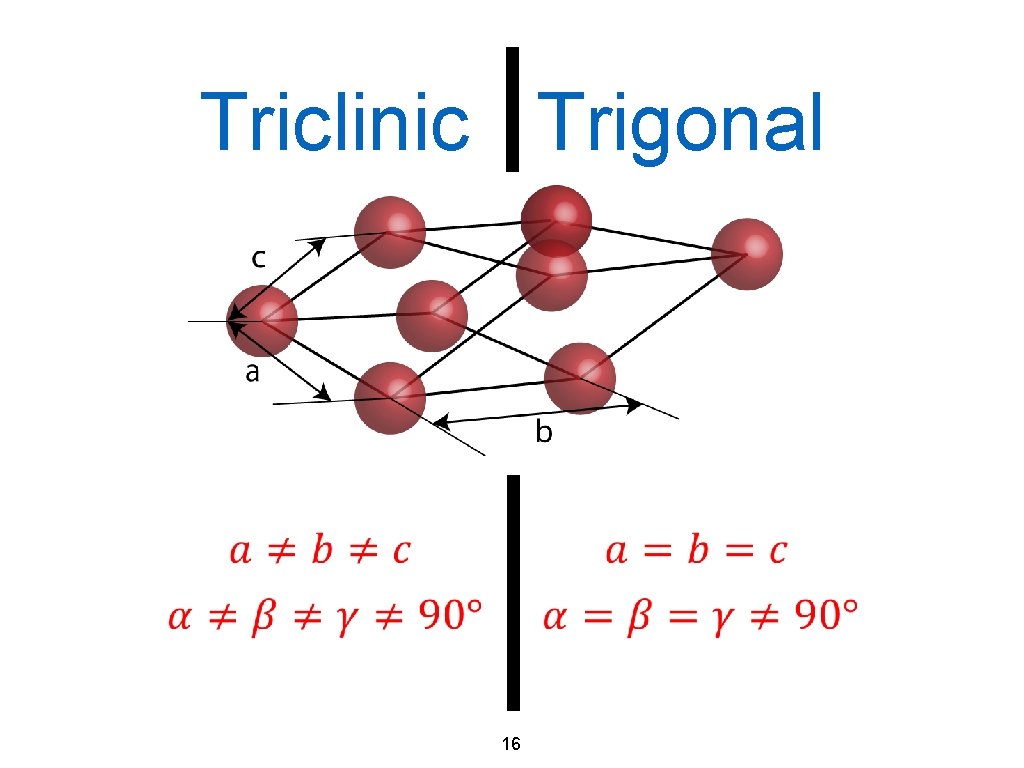
Triclinic Trigonal 16
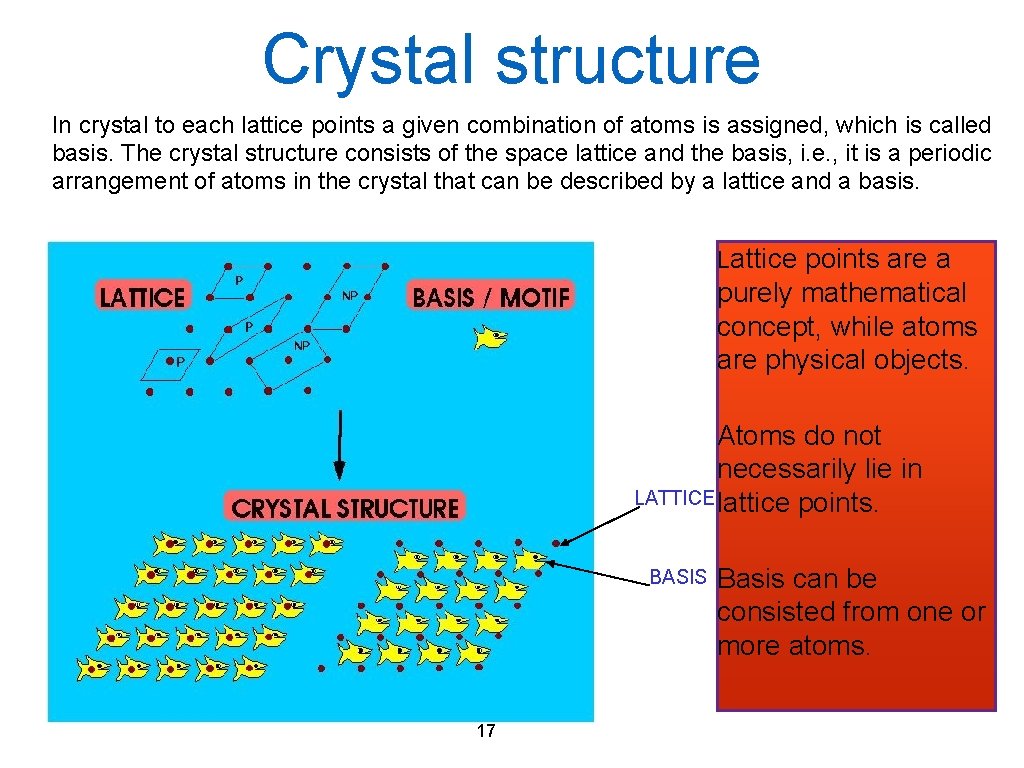
Crystal structure In crystal to each lattice points a given combination of atoms is assigned, which is called basis. The crystal structure consists of the space lattice and the basis, i. e. , it is a periodic arrangement of atoms in the crystal that can be described by a lattice and a basis. Lattice points are a purely mathematical concept, while atoms are physical objects. Atoms do not necessarily lie in LATTICE lattice points. BASIS 17 Basis can be consisted from one or more atoms.
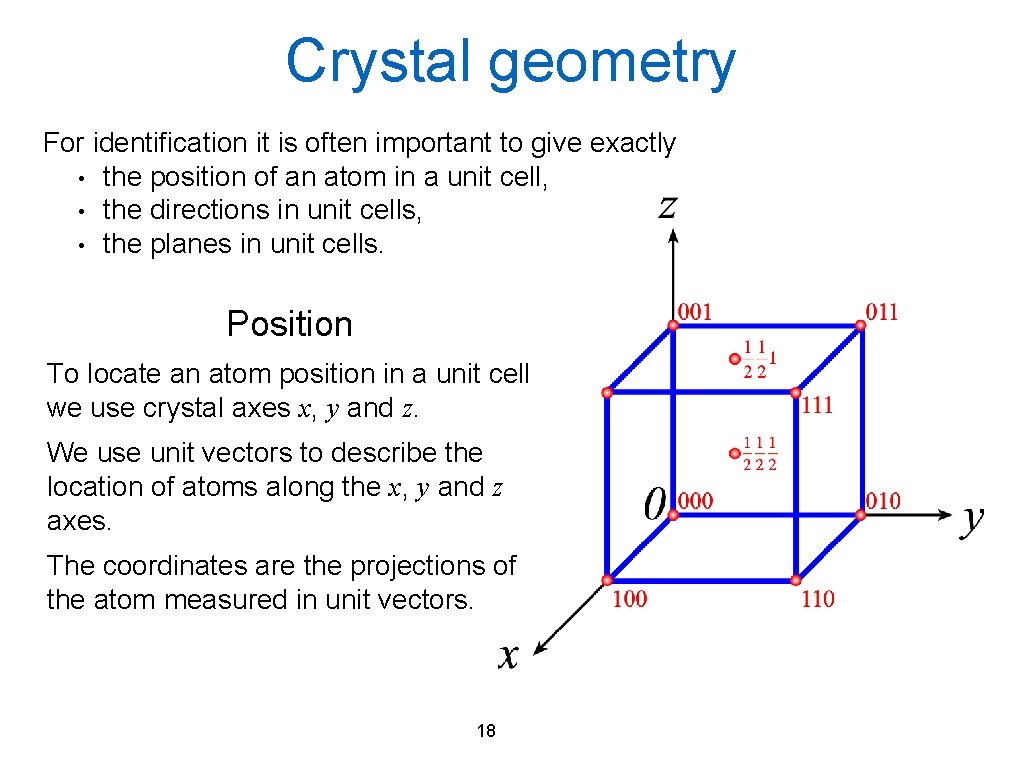
Crystal geometry For identification it is often important to give exactly • the position of an atom in a unit cell, • the directions in unit cells, • the planes in unit cells. Position To locate an atom position in a unit cell we use crystal axes x, y and z. We use unit vectors to describe the location of atoms along the x, y and z axes. The coordinates are the projections of the atom measured in unit vectors. 18
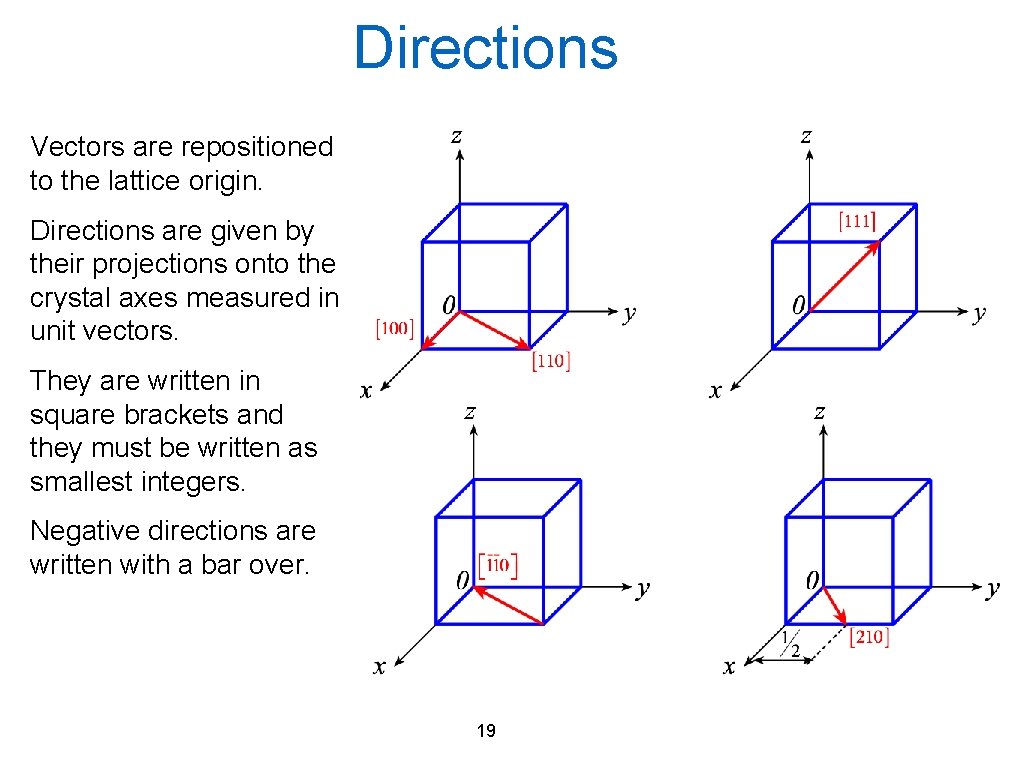
Directions Vectors are repositioned to the lattice origin. Directions are given by their projections onto the crystal axes measured in unit vectors. They are written in square brackets and they must be written as smallest integers. Negative directions are written with a bar over. 19
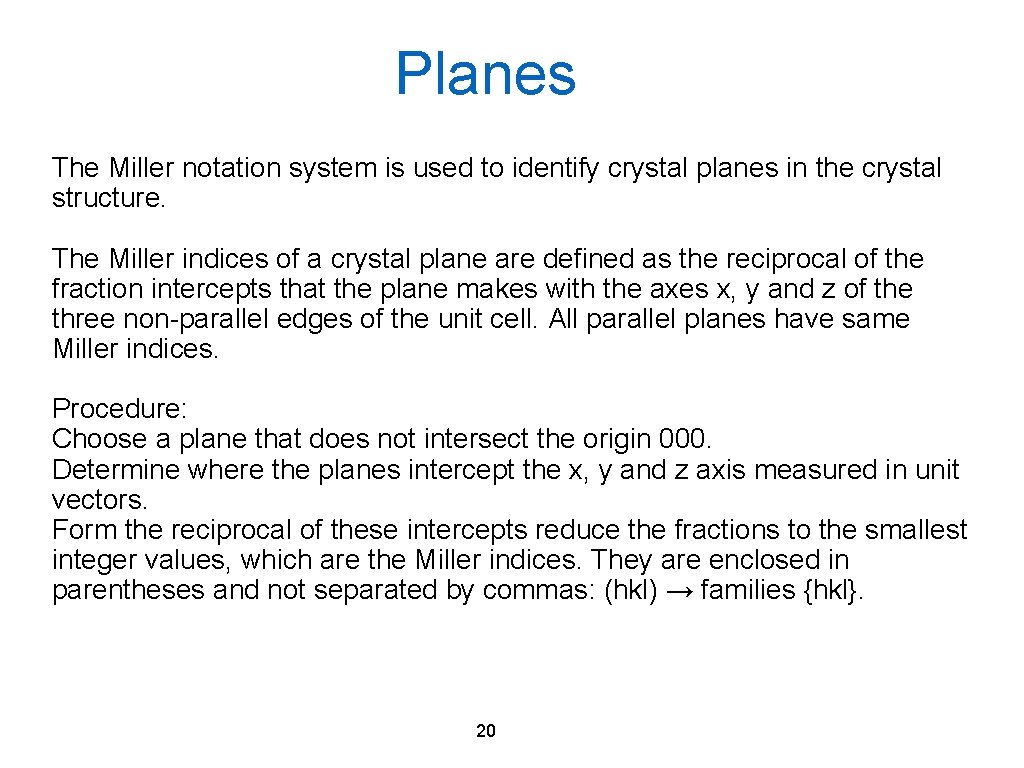
Planes The Miller notation system is used to identify crystal planes in the crystal structure. The Miller indices of a crystal plane are defined as the reciprocal of the fraction intercepts that the plane makes with the axes x, y and z of the three non-parallel edges of the unit cell. All parallel planes have same Miller indices. Procedure: Choose a plane that does not intersect the origin 000. Determine where the planes intercept the x, y and z axis measured in unit vectors. Form the reciprocal of these intercepts reduce the fractions to the smallest integer values, which are the Miller indices. They are enclosed in parentheses and not separated by commas: (hkl) → families {hkl}. 20
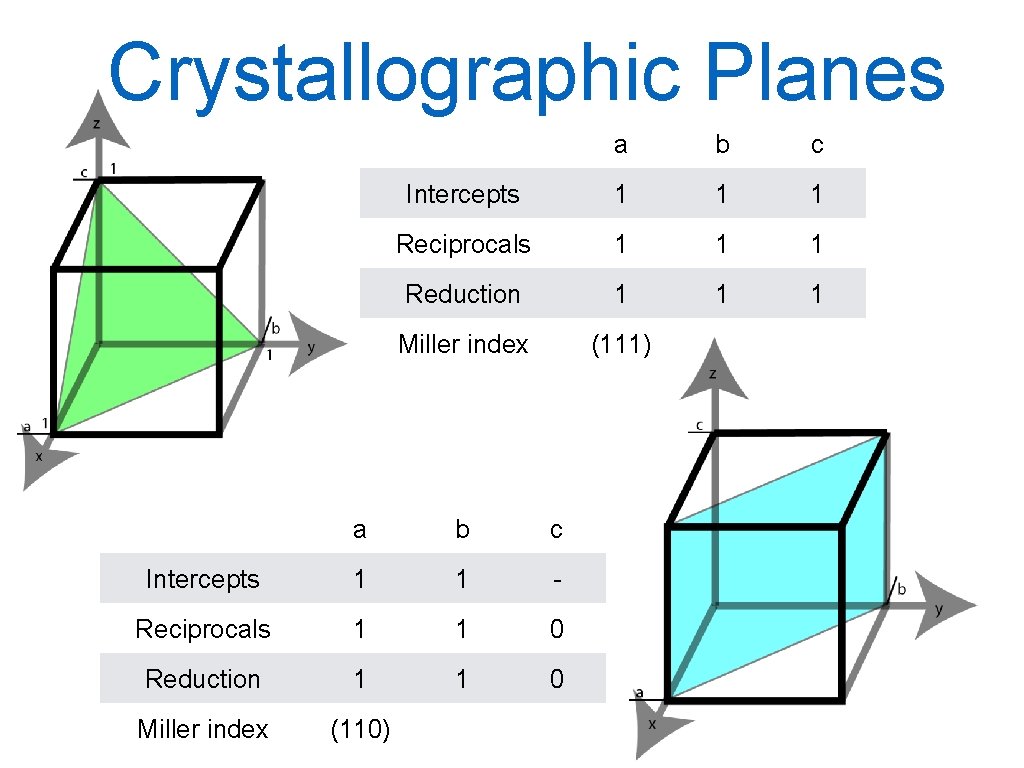
Crystallographic Planes a b c Intercepts 1 1 1 Reciprocals 1 1 1 Reduction 1 1 1 Miller index (111) a b c Intercepts 1 1 - Reciprocals 1 1 0 Reduction 1 1 0 Miller index (110) 21
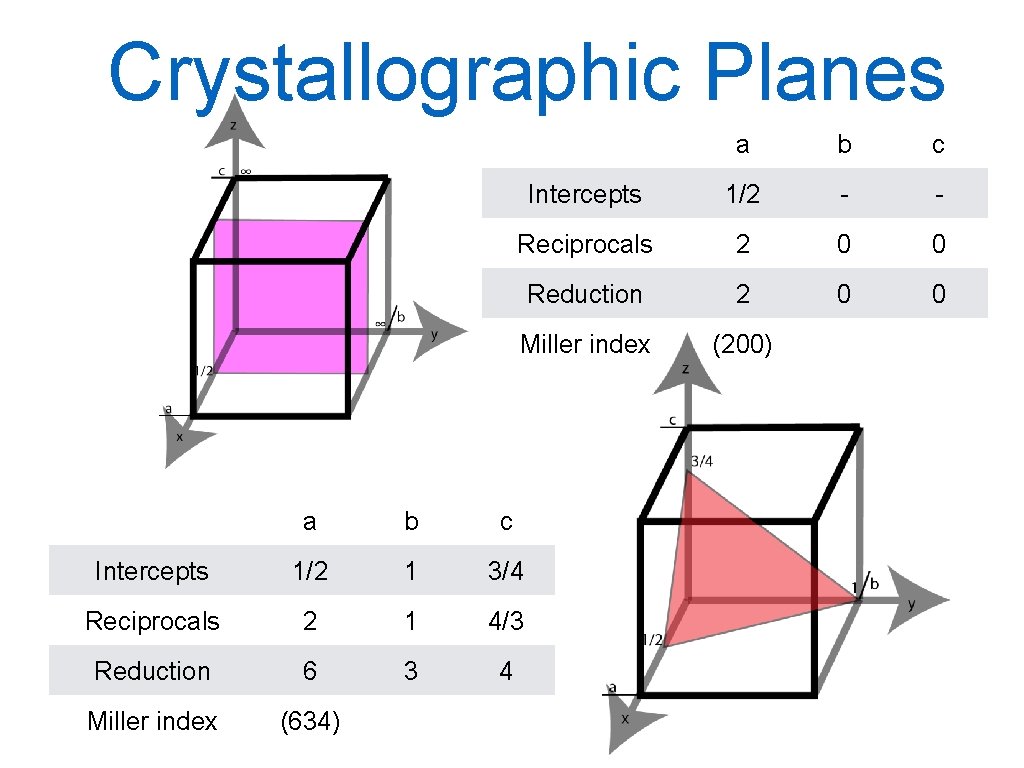
Crystallographic Planes a b c Intercepts 1/2 - - Reciprocals 2 0 0 Reduction 2 0 0 Miller index (200) a b c Intercepts 1/2 1 3/4 Reciprocals 2 1 4/3 Reduction 6 3 4 Miller index (634) 22
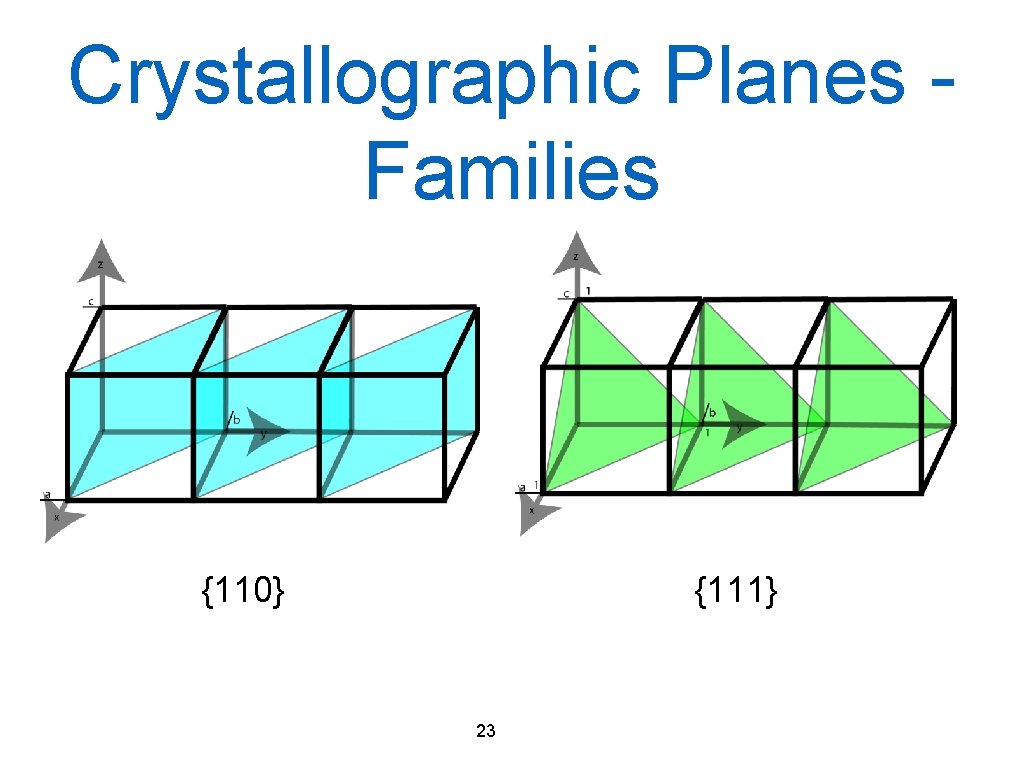
Crystallographic Planes Families {110} {111} 23
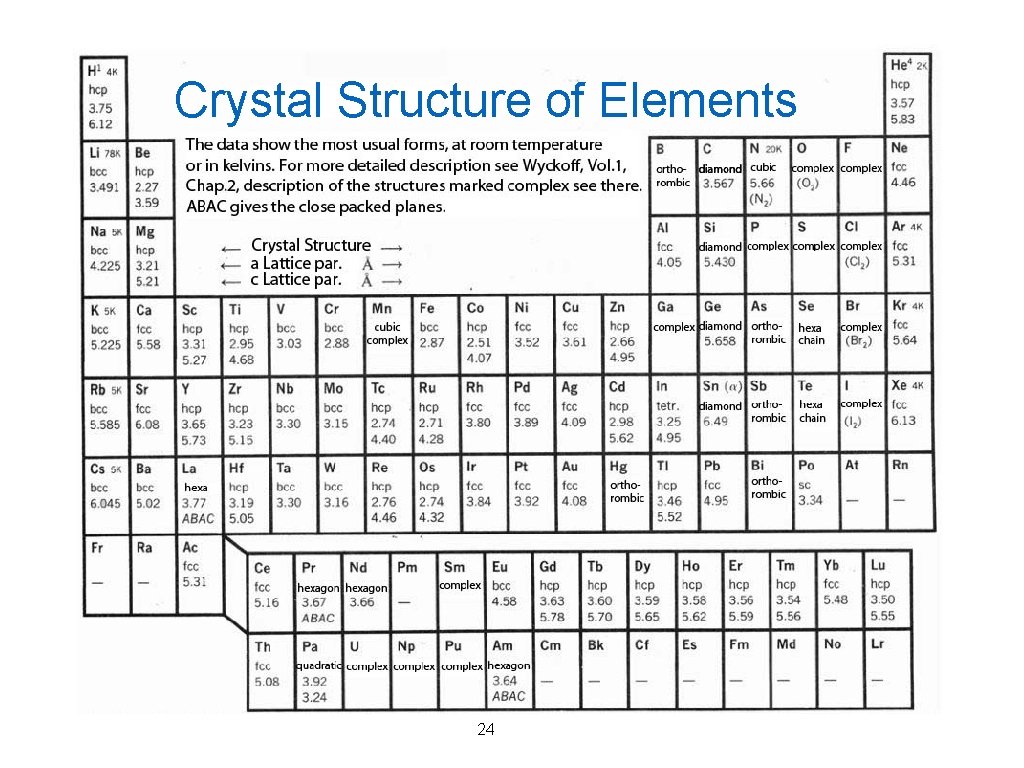
Crystal Structure of Elements 24
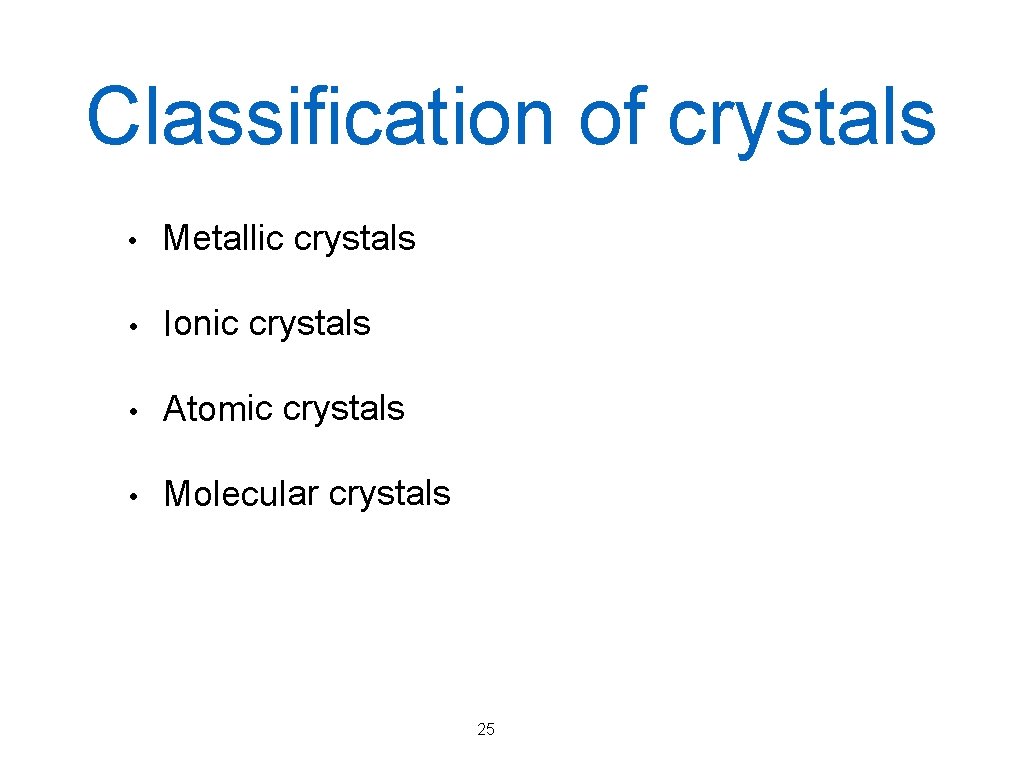
Classification of crystals • Metallic crystals • Ionic crystals • Atomic crystals • Molecular crystals 25
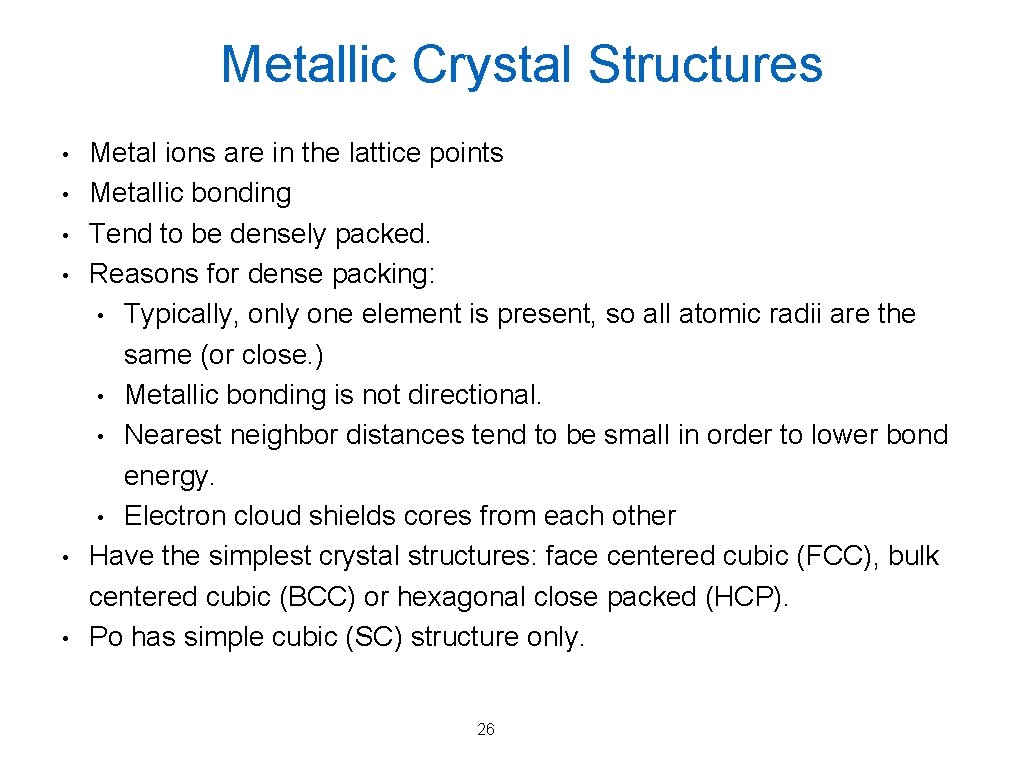
Metallic Crystal Structures • • • Metal ions are in the lattice points Metallic bonding Tend to be densely packed. Reasons for dense packing: • Typically, only one element is present, so all atomic radii are the same (or close. ) • Metallic bonding is not directional. • Nearest neighbor distances tend to be small in order to lower bond energy. • Electron cloud shields cores from each other Have the simplest crystal structures: face centered cubic (FCC), bulk centered cubic (BCC) or hexagonal close packed (HCP). Po has simple cubic (SC) structure only. 26
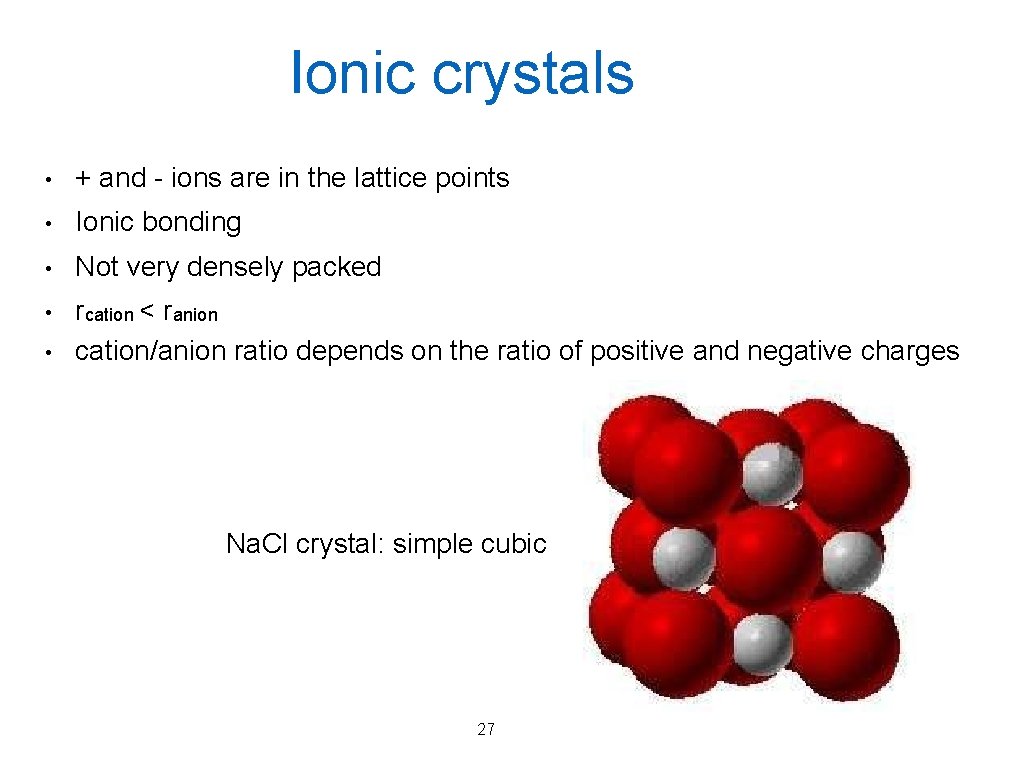
Ionic crystals • + and - ions are in the lattice points • Ionic bonding • Not very densely packed • rcation < ranion cation/anion ratio depends on the ratio of positive and negative charges • Na. Cl crystal: simple cubic 27
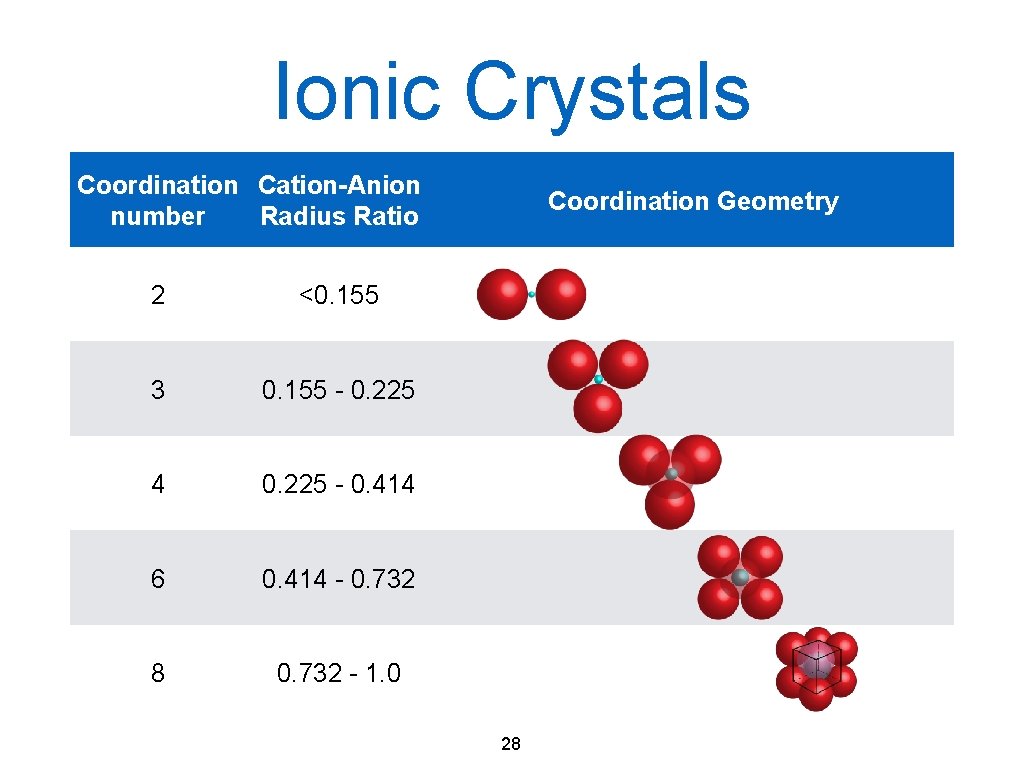
Ionic Crystals Coordination Cation-Anion number Radius Ratio 2 <0. 155 3 0. 155 - 0. 225 4 0. 225 - 0. 414 6 0. 414 - 0. 732 8 0. 732 - 1. 0 Coordination Geometry 28
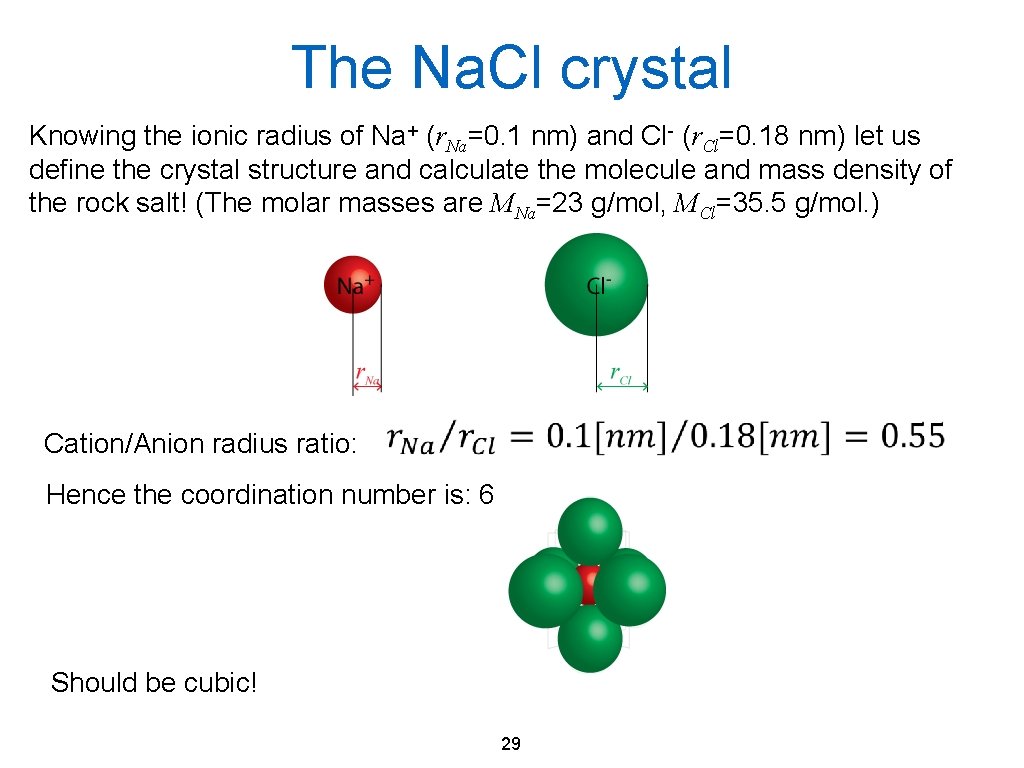
The Na. Cl crystal Knowing the ionic radius of Na+ (r. Na=0. 1 nm) and Cl- (r. Cl=0. 18 nm) let us define the crystal structure and calculate the molecule and mass density of the rock salt! (The molar masses are MNa=23 g/mol, MCl=35. 5 g/mol. ) Cation/Anion radius ratio: Hence the coordination number is: 6 Should be cubic! 29
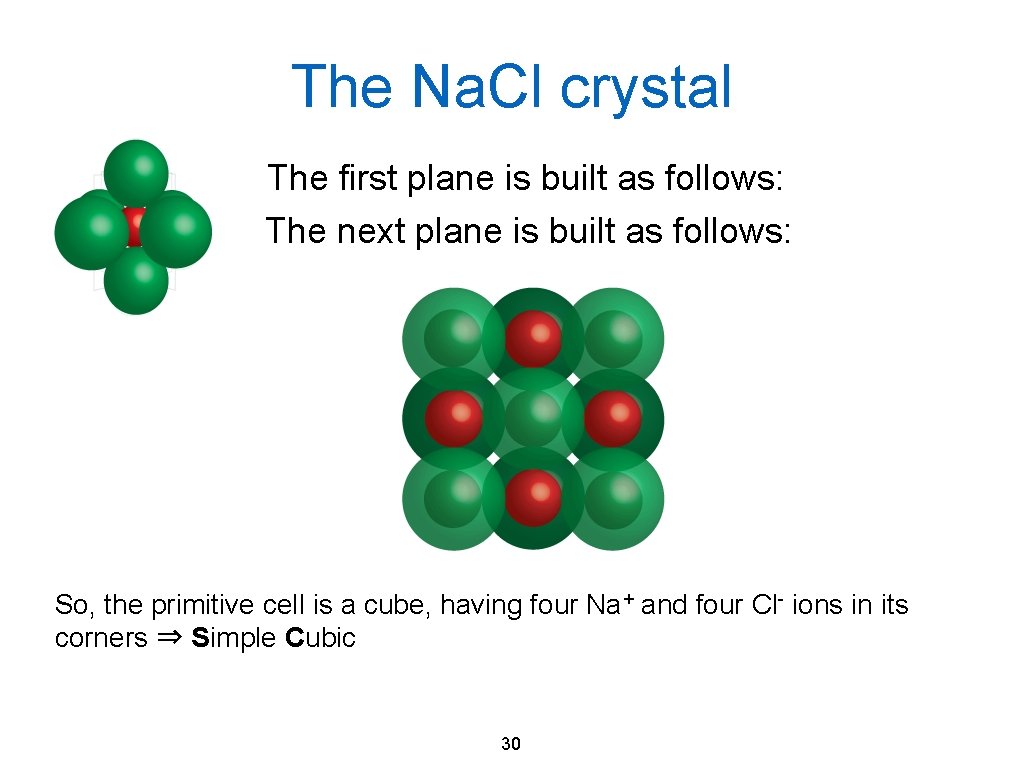
The Na. Cl crystal The first plane is built as follows: The next plane is built as follows: So, the primitive cell is a cube, having four Na+ and four Cl- ions in its corners ⇒ Simple Cubic 30
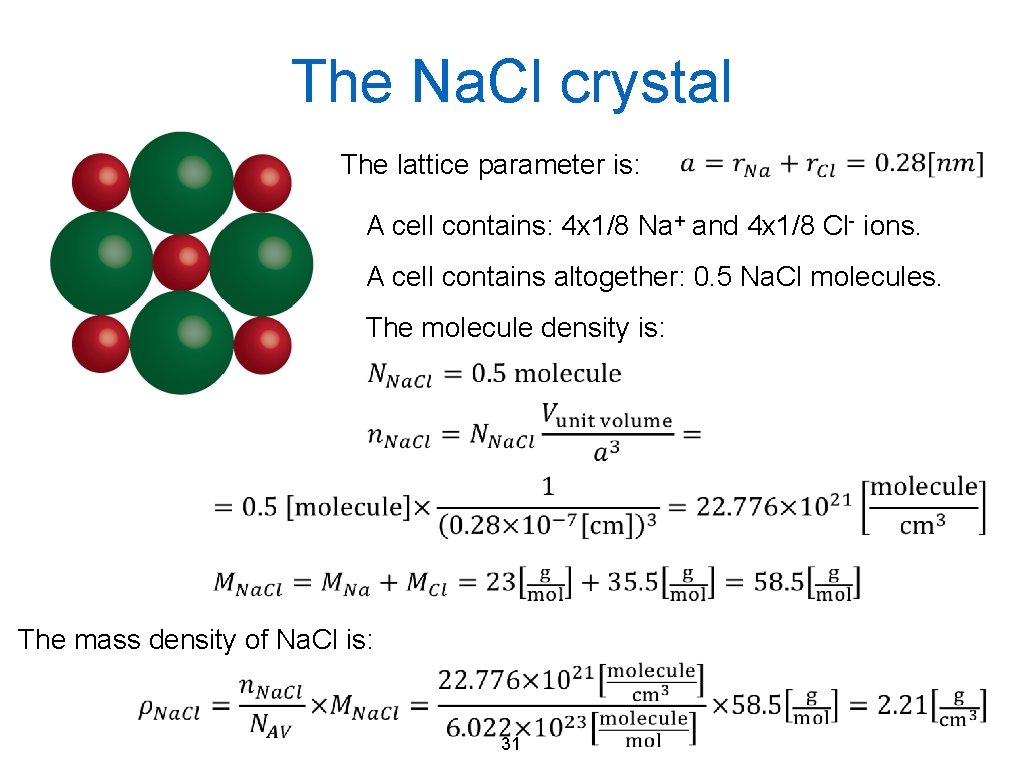
The Na. Cl crystal The lattice parameter is: A cell contains: 4 x 1/8 Na+ and 4 x 1/8 Cl- ions. A cell contains altogether: 0. 5 Na. Cl molecules. The molecule density is: The mass density of Na. Cl is: 31
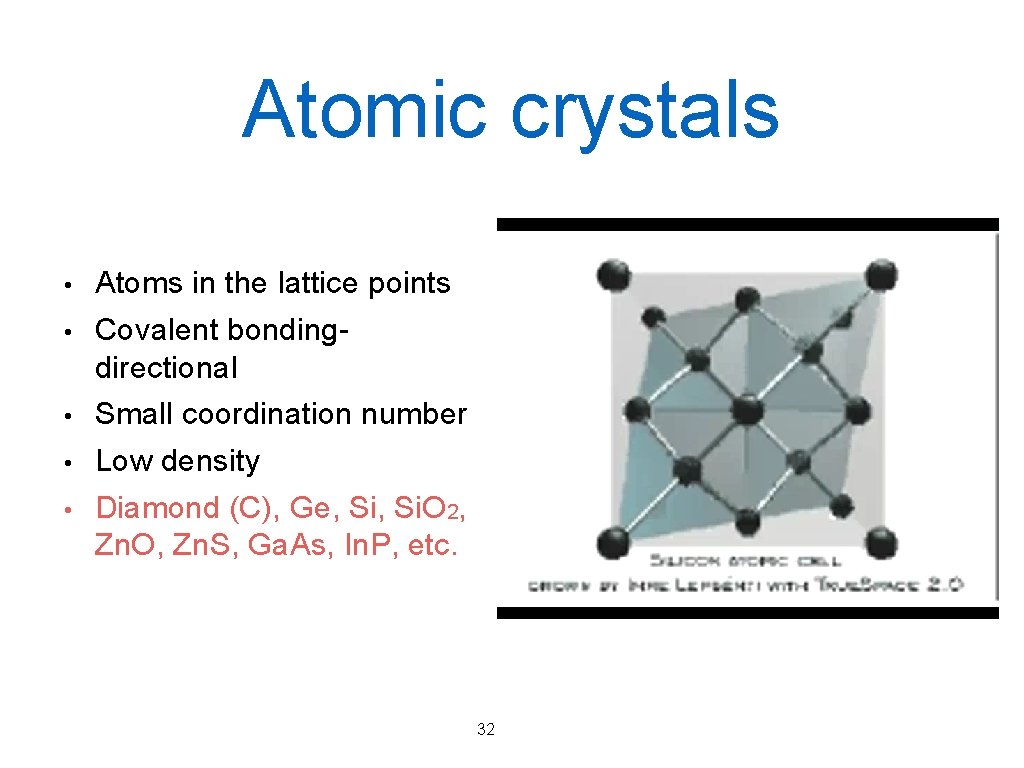
Atomic crystals • • • Atoms in the lattice points Covalent bondingdirectional Small coordination number Low density Diamond (C), Ge, Si. O 2, Zn. O, Zn. S, Ga. As, In. P, etc. 32
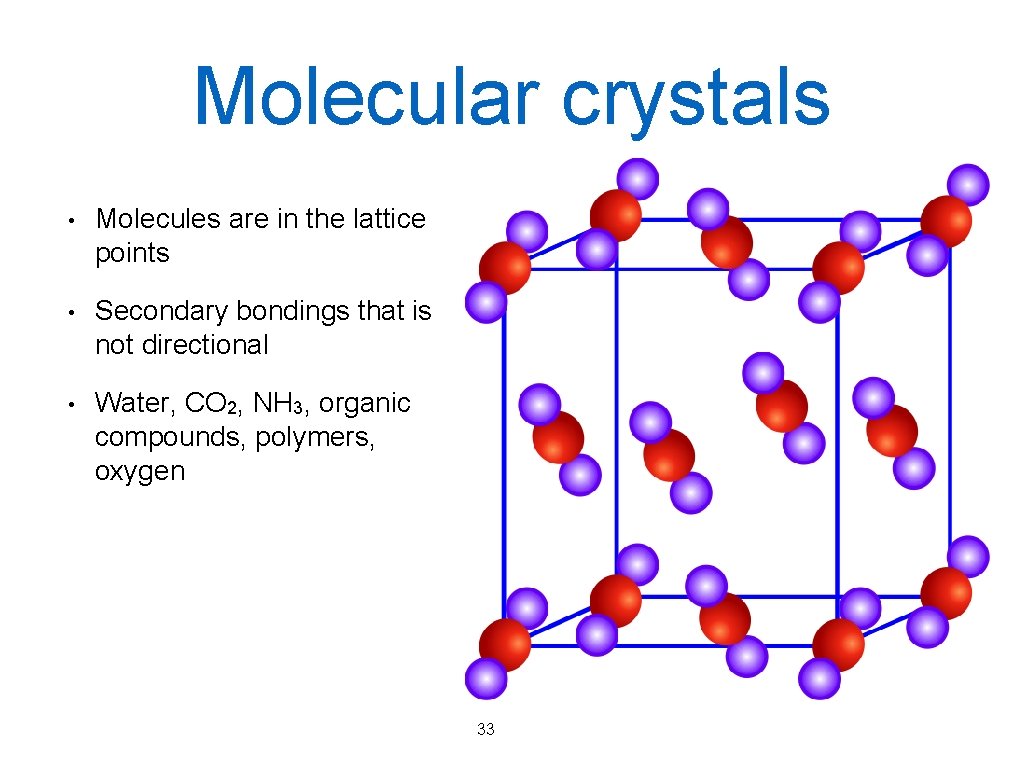
Molecular crystals • Molecules are in the lattice points • Secondary bondings that is not directional • Water, CO 2, NH 3, organic compounds, polymers, oxygen 33
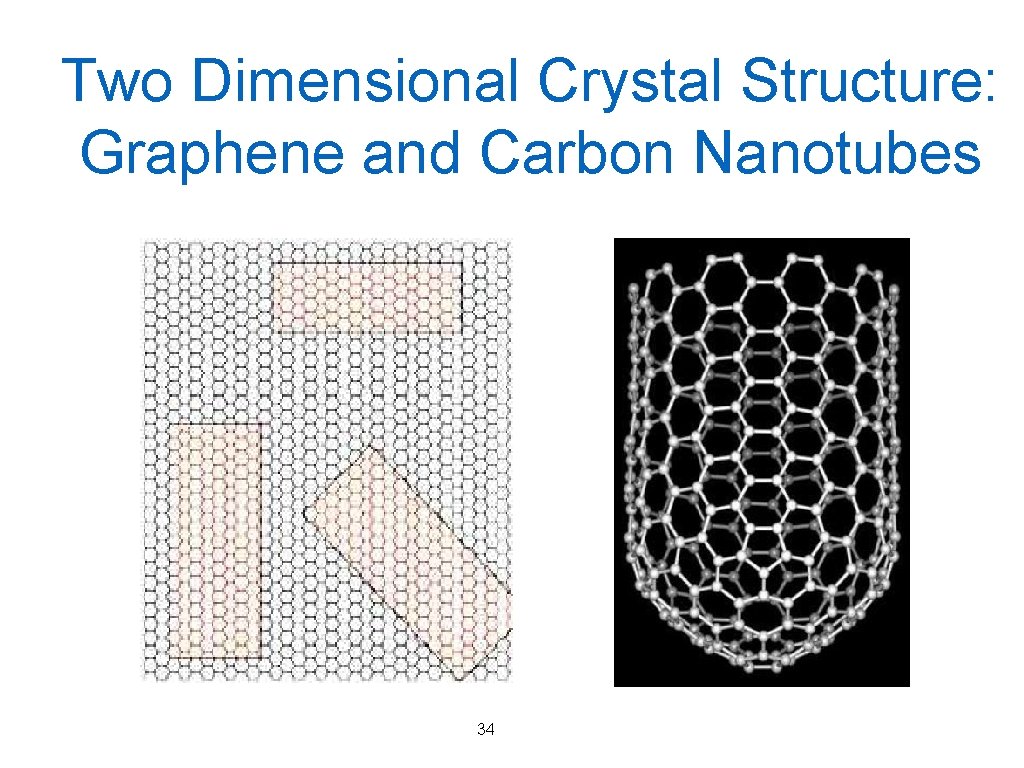
Two Dimensional Crystal Structure: Graphene and Carbon Nanotubes 34
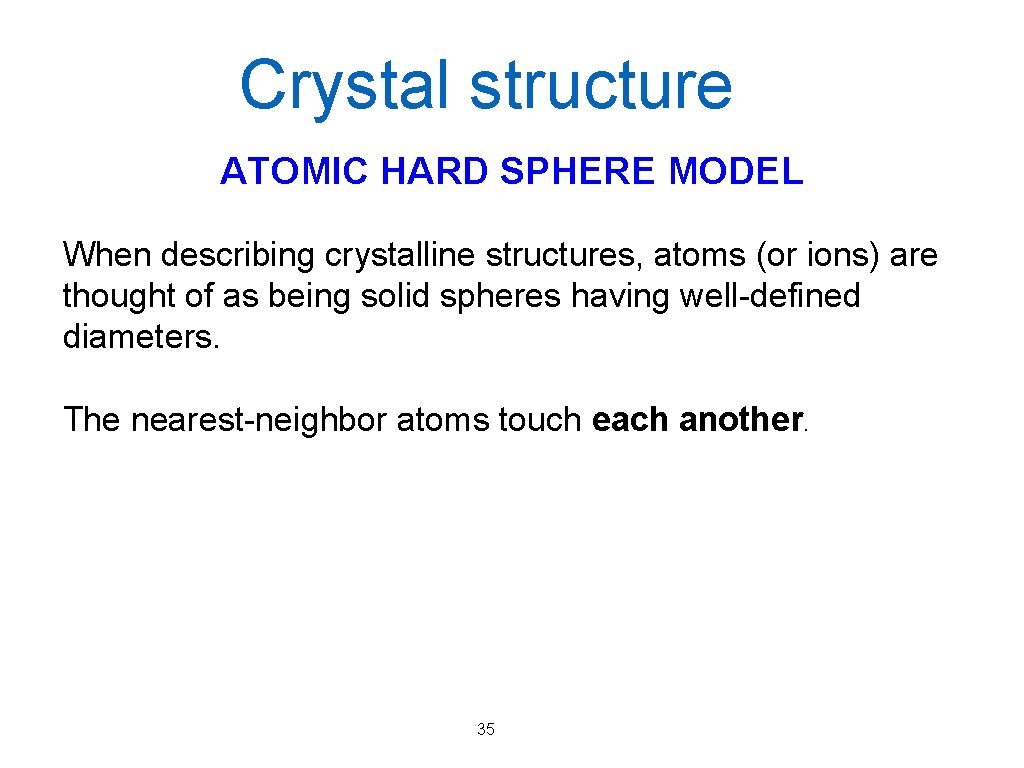
Crystal structure ATOMIC HARD SPHERE MODEL When describing crystalline structures, atoms (or ions) are thought of as being solid spheres having well-defined diameters. The nearest-neighbor atoms touch each another. 35
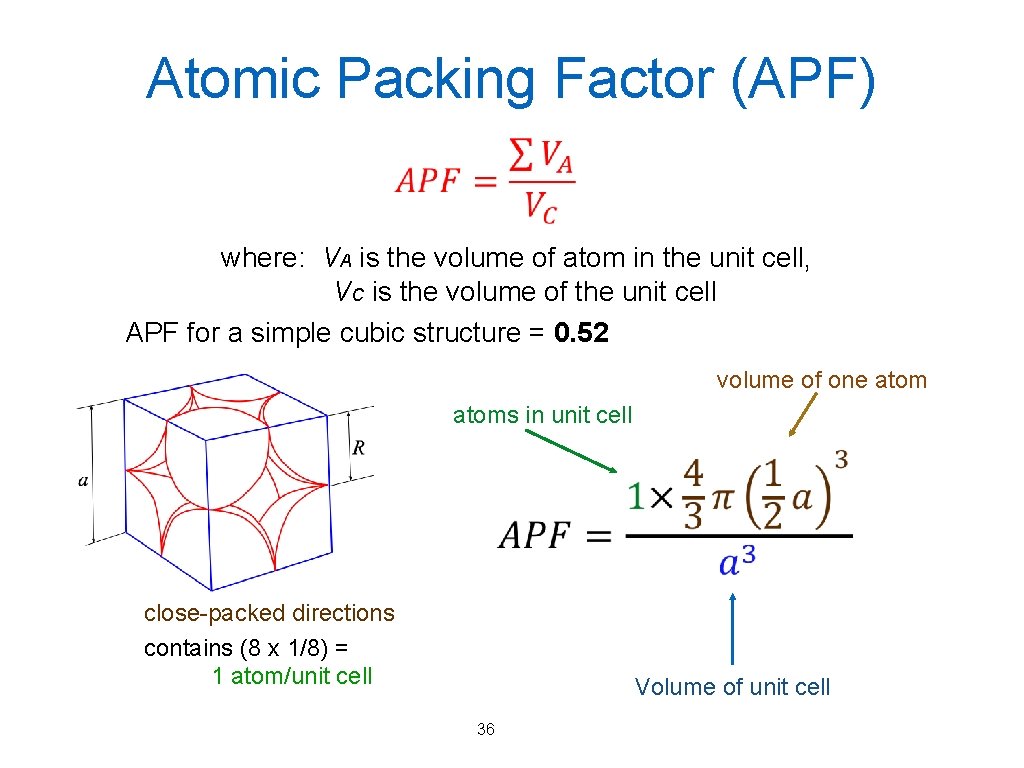
Atomic Packing Factor (APF) where: VA is the volume of atom in the unit cell, VC is the volume of the unit cell APF for a simple cubic structure = 0. 52 volume of one atoms in unit cell close-packed directions contains (8 x 1/8) = 1 atom/unit cell Volume of unit cell 36
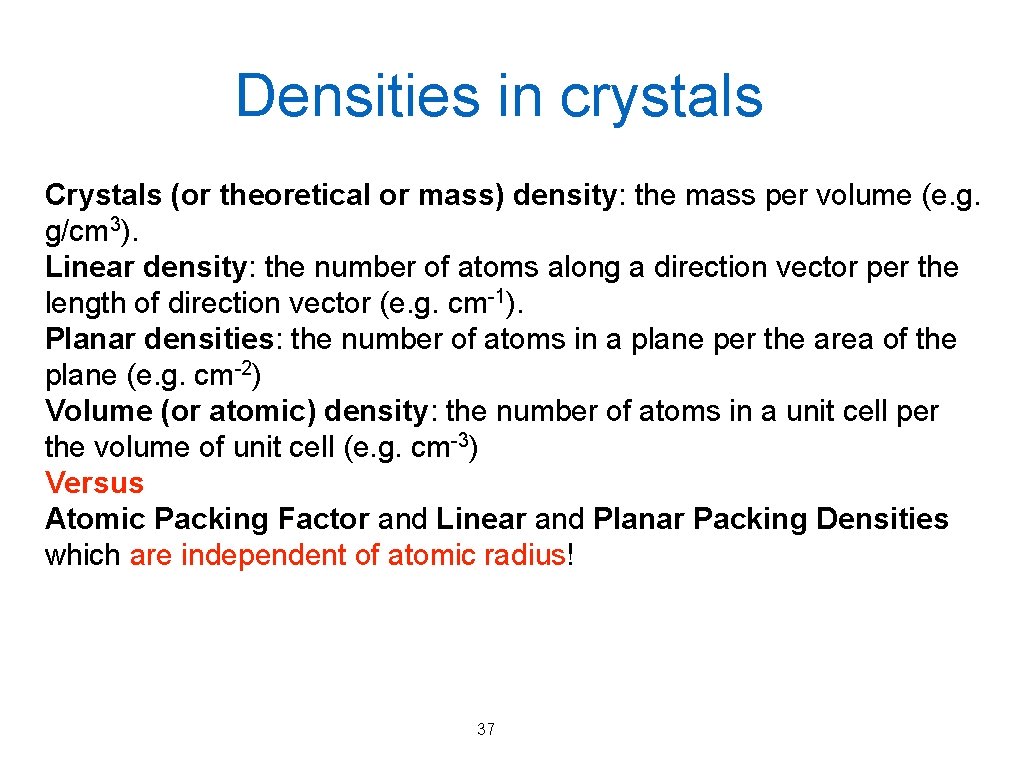
Densities in crystals Crystals (or theoretical or mass) density: the mass per volume (e. g. g/cm 3). Linear density: the number of atoms along a direction vector per the length of direction vector (e. g. cm-1). Planar densities: the number of atoms in a plane per the area of the plane (e. g. cm-2) Volume (or atomic) density: the number of atoms in a unit cell per the volume of unit cell (e. g. cm-3) Versus Atomic Packing Factor and Linear and Planar Packing Densities which are independent of atomic radius! 37
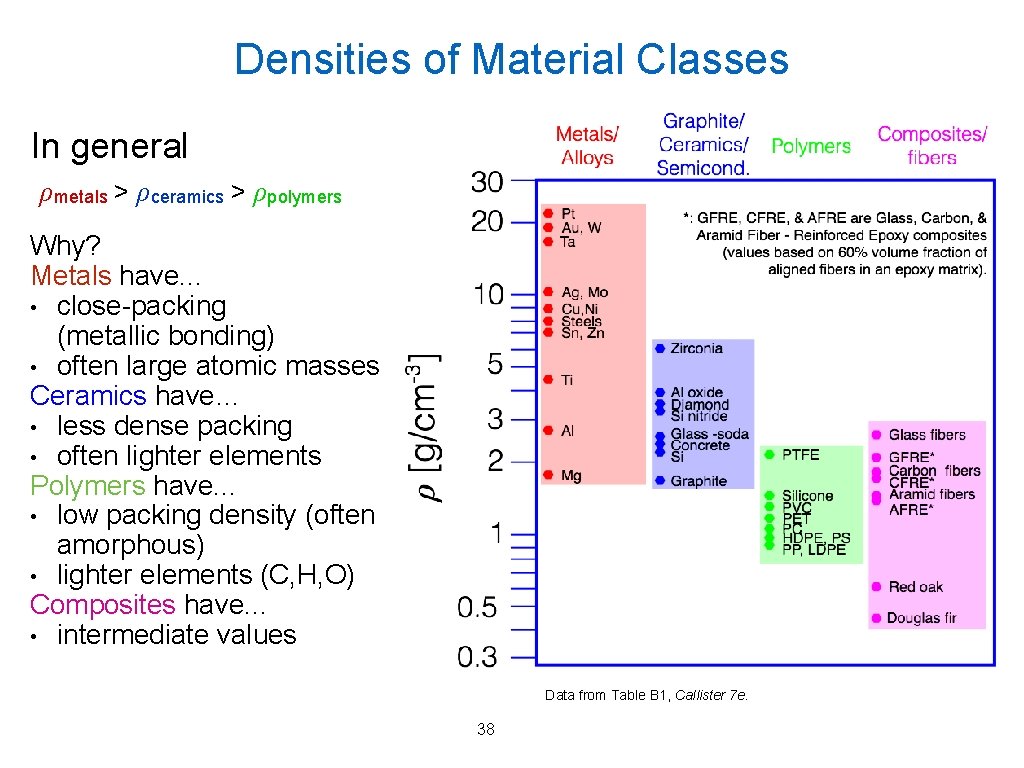
Densities of Material Classes In general ρmetals > ρceramics > ρpolymers Why? Metals have. . . • close-packing (metallic bonding) • often large atomic masses Ceramics have… • less dense packing • often lighter elements Polymers have. . . • low packing density (often amorphous) • lighter elements (C, H, O) Composites have. . . • intermediate values Data from Table B 1, Callister 7 e. 38
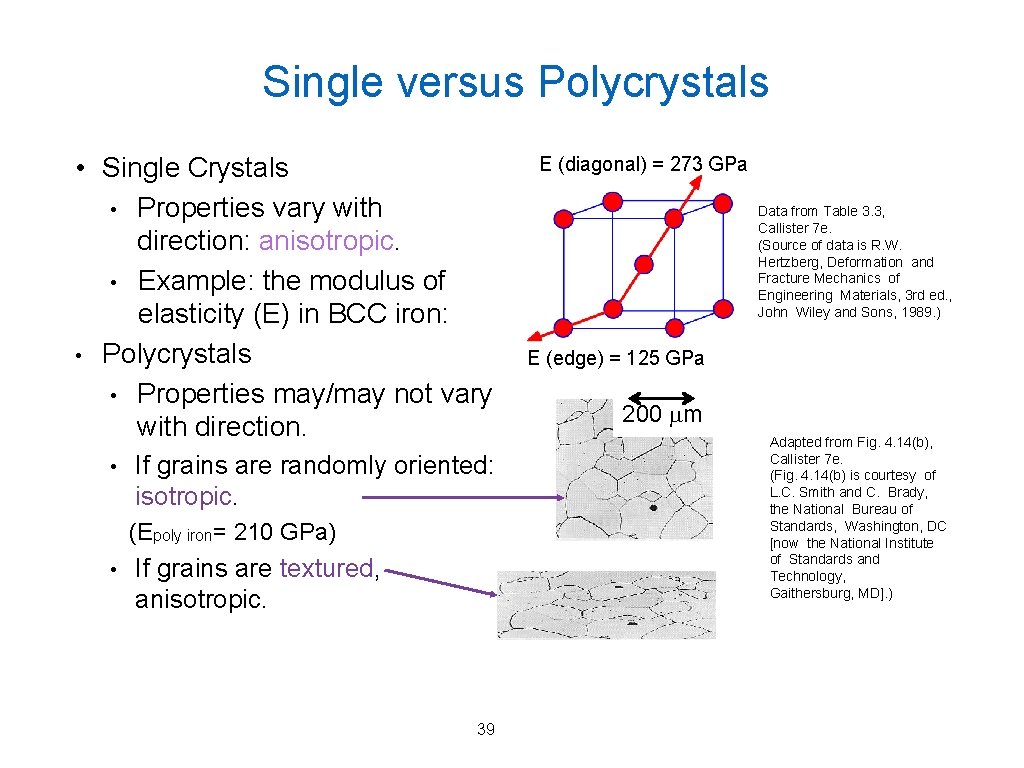
Single versus Polycrystals • Single Crystals • Properties vary with direction: anisotropic. • Example: the modulus of elasticity (E) in BCC iron: • Polycrystals • Properties may/may not vary with direction. • If grains are randomly oriented: isotropic. (Epoly iron= 210 GPa) • If grains are textured, anisotropic. 39 E (diagonal) = 273 GPa Data from Table 3. 3, Callister 7 e. (Source of data is R. W. Hertzberg, Deformation and Fracture Mechanics of Engineering Materials, 3 rd ed. , John Wiley and Sons, 1989. ) E (edge) = 125 GPa 200 mm Adapted from Fig. 4. 14(b), Callister 7 e. (Fig. 4. 14(b) is courtesy of L. C. Smith and C. Brady, the National Bureau of Standards, Washington, DC [now the National Institute of Standards and Technology, Gaithersburg, MD]. )
![Effects of Anisotropy Modulus of Elasticity GPa Metal Structure 100 111 Aluminum FCC 63 Effects of Anisotropy: Modulus of Elasticity (GPa) Metal Structure [100] [111] Aluminum FCC 63.](https://slidetodoc.com/presentation_image_h2/ee3cdeade56cc9d8b89c2d84a5f2ebb2/image-40.jpg)
Effects of Anisotropy: Modulus of Elasticity (GPa) Metal Structure [100] [111] Aluminum FCC 63. 7 72. 6 76. 1 Copper FCC 66. 7 130. 3 191. 1 Iron BCC 125. 0 210. 5 272. 7 Tungsten BCC 384. 6 Based on: R. W. Hertzberg, Deformation and Fracture Mechanics of Engineering Materials, 3 rd. edition, 1989. John Wiley & Sons, New York 40
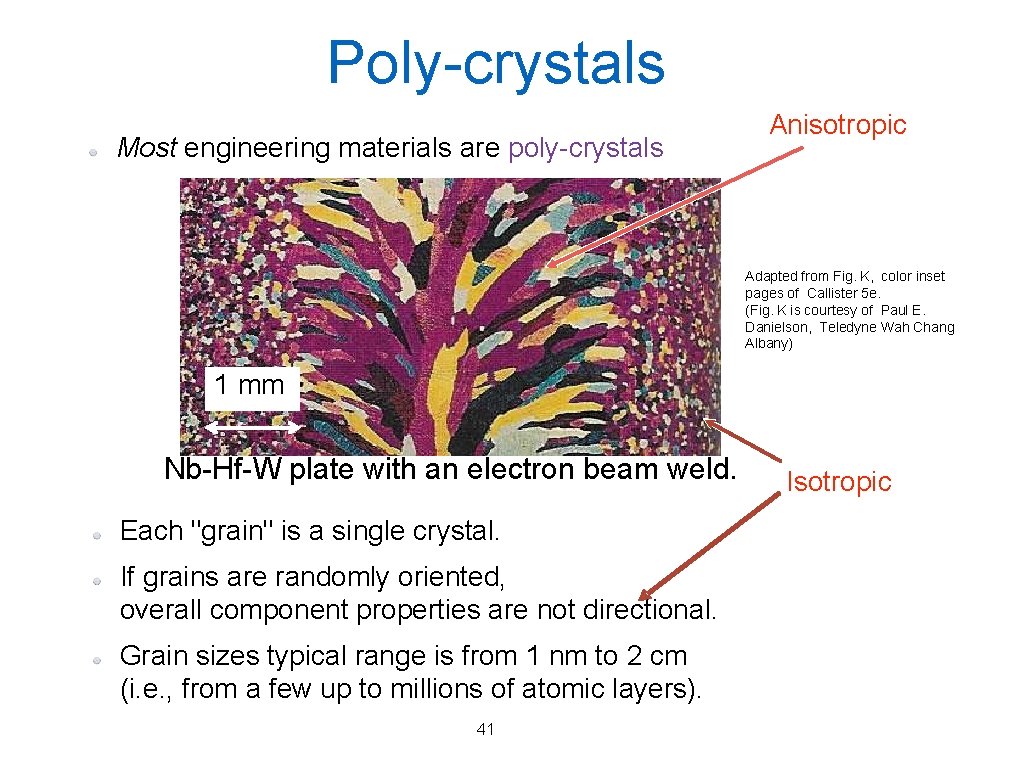
Poly-crystals Most engineering materials are poly-crystals Anisotropic Adapted from Fig. K, color inset pages of Callister 5 e. (Fig. K is courtesy of Paul E. Danielson, Teledyne Wah Chang Albany) 1 mm Nb-Hf-W plate with an electron beam weld. Each "grain" is a single crystal. If grains are randomly oriented, overall component properties are not directional. Grain sizes typical range is from 1 nm to 2 cm (i. e. , from a few up to millions of atomic layers). 41 Isotropic
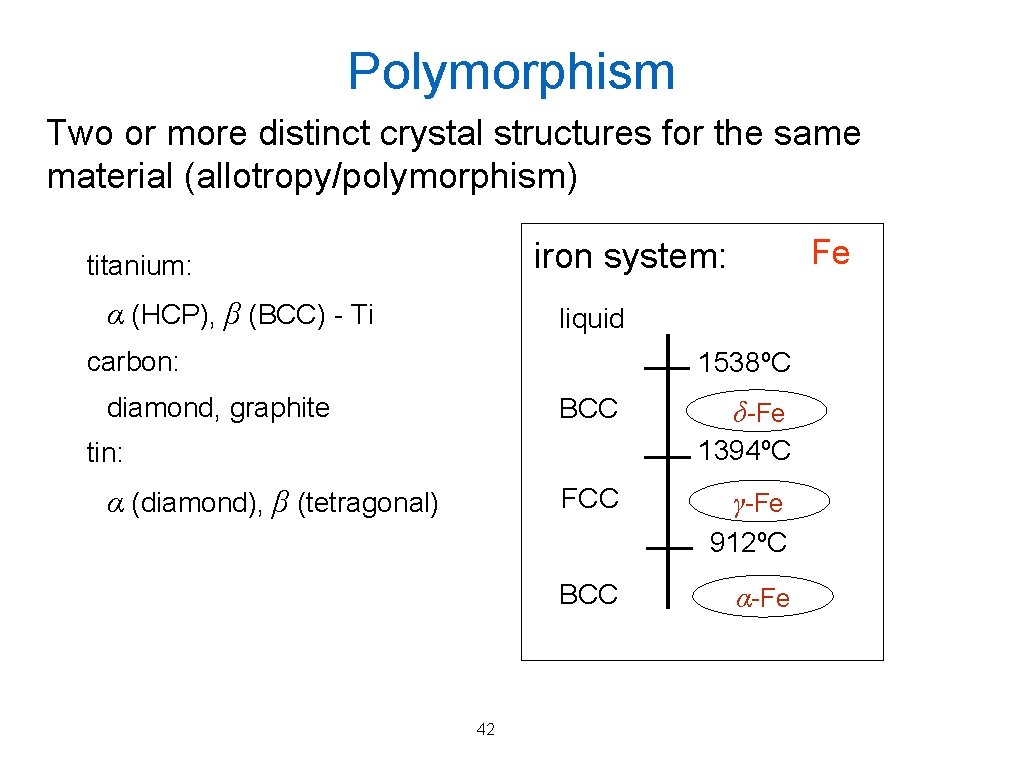
Polymorphism Two or more distinct crystal structures for the same material (allotropy/polymorphism) Fe iron system: titanium: α (HCP), β (BCC) - Ti liquid carbon: 1538ºC diamond, graphite BCC δ-Fe 1394ºC tin: FCC α (diamond), β (tetragonal) γ-Fe 912ºC BCC 42 α-Fe
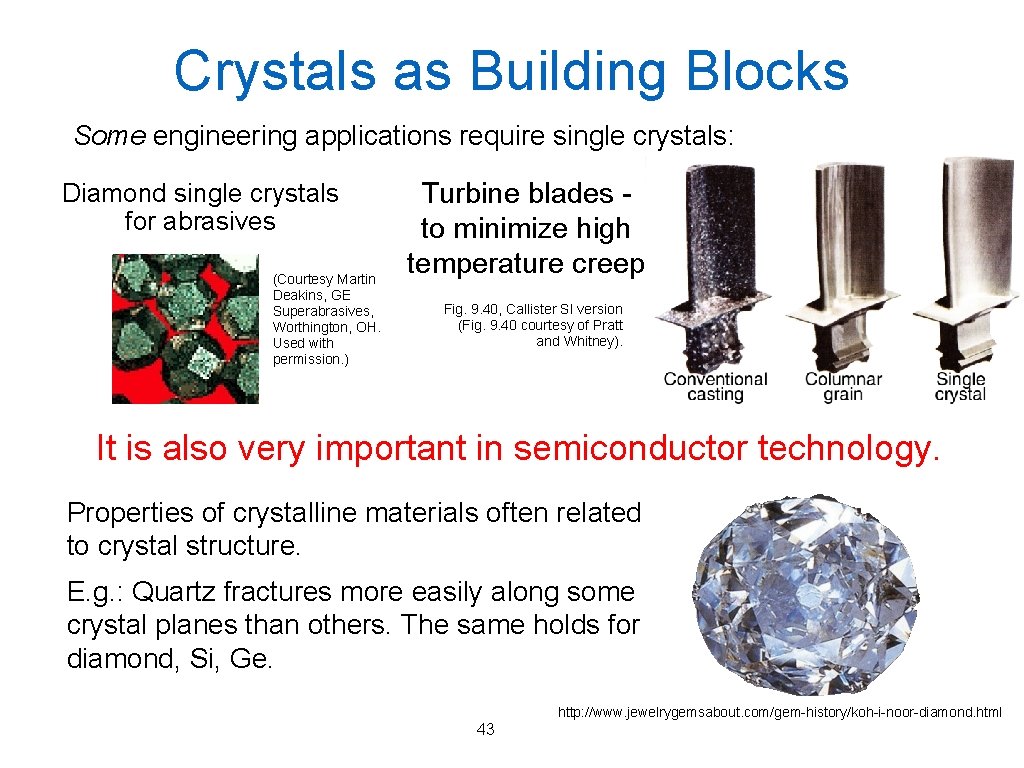
Crystals as Building Blocks Some engineering applications require single crystals: Diamond single crystals for abrasives (Courtesy Martin Deakins, GE Superabrasives, Worthington, OH. Used with permission. ) Turbine blades to minimize high temperature creep Fig. 9. 40, Callister SI version (Fig. 9. 40 courtesy of Pratt and Whitney). It is also very important in semiconductor technology. Properties of crystalline materials often related to crystal structure. E. g. : Quartz fractures more easily along some crystal planes than others. The same holds for diamond, Si, Ge. 43 http: //www. jewelrygemsabout. com/gem-history/koh-i-noor-diamond. html
Crystal solid and amorphous solid
Nonliving solid with crystal like properties
Homologous
Honors its atomic
Example of solid solution?
Covalent network solid vs molecular solid
Polycrystalline solids
Crystalline solid and amorphous solid
Is cotton candy anisotropic
When a solid completely penetrates another solid
Interpenetration of surfaces
Examples of separation by evaporation
Definition of crystalline
Four basic waiting line structures
Oo solid
Standard dry/solid measuring cups come in what four sizes
What are the outer planets mostly made of
What 2/3 cup
Hình ảnh bộ gõ cơ thể búng tay
Frameset trong html5
Bổ thể
Tỉ lệ cơ thể trẻ em
Gấu đi như thế nào
Tư thế worm breton
Alleluia hat len nguoi oi
Môn thể thao bắt đầu bằng chữ đua
Thế nào là hệ số cao nhất
Các châu lục và đại dương trên thế giới
Cong thức tính động năng
Trời xanh đây là của chúng ta thể thơ
Mật thư anh em như thể tay chân
Làm thế nào để 102-1=99
độ dài liên kết
Các châu lục và đại dương trên thế giới
Thể thơ truyền thống
Quá trình desamine hóa có thể tạo ra
Một số thể thơ truyền thống
Cái miệng xinh xinh thế chỉ nói điều hay thôi
Vẽ hình chiếu vuông góc của vật thể sau
Nguyên nhân của sự mỏi cơ sinh 8
đặc điểm cơ thể của người tối cổ
Thế nào là giọng cùng tên
Vẽ hình chiếu đứng bằng cạnh của vật thể
Tia chieu sa te