Macromolecules Macromolecules Large molecules greater than 100 000
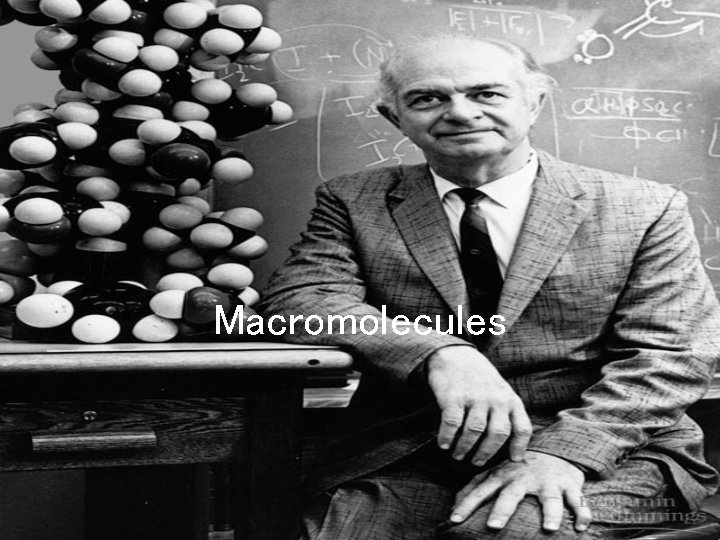
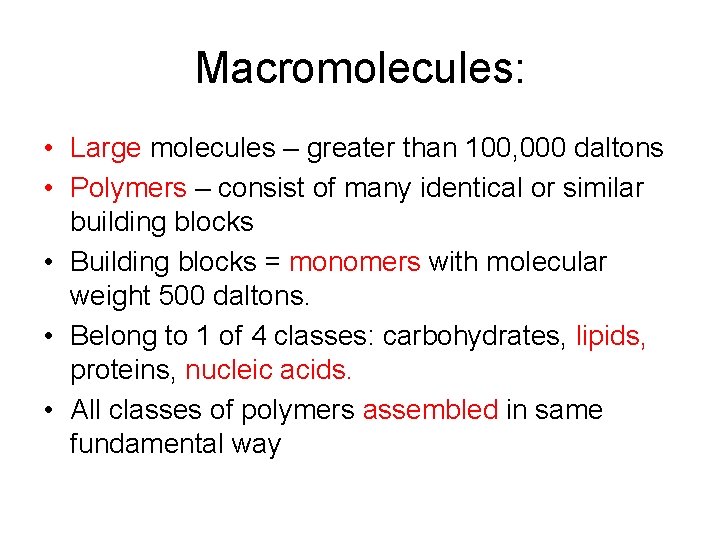
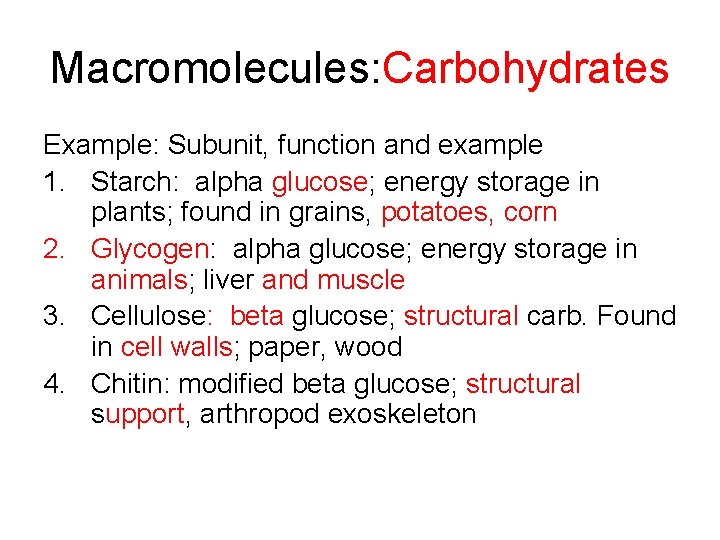
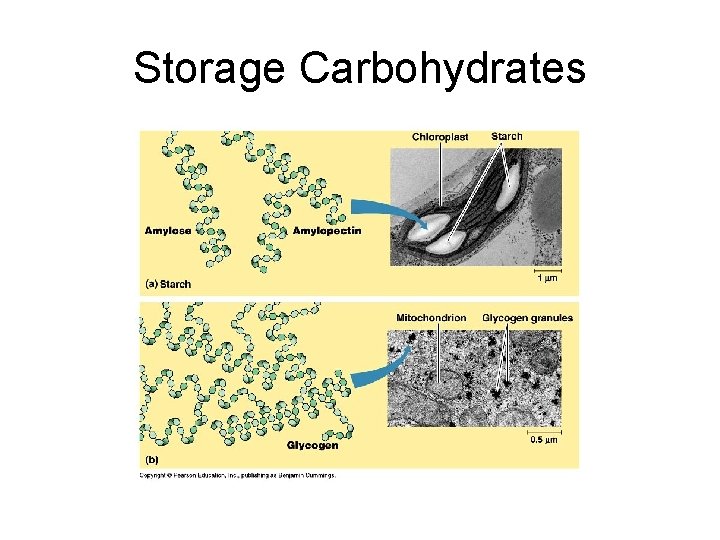
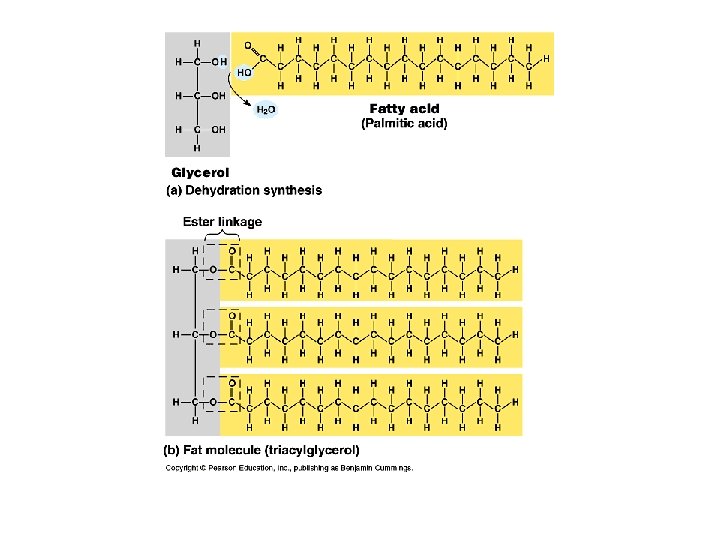
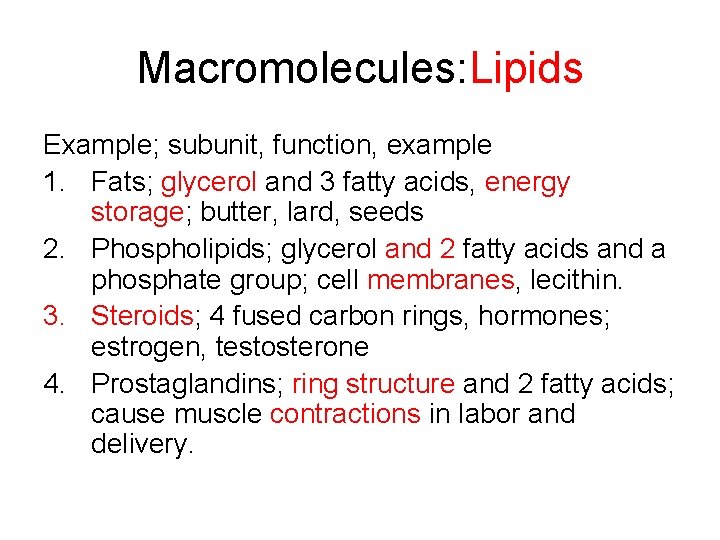
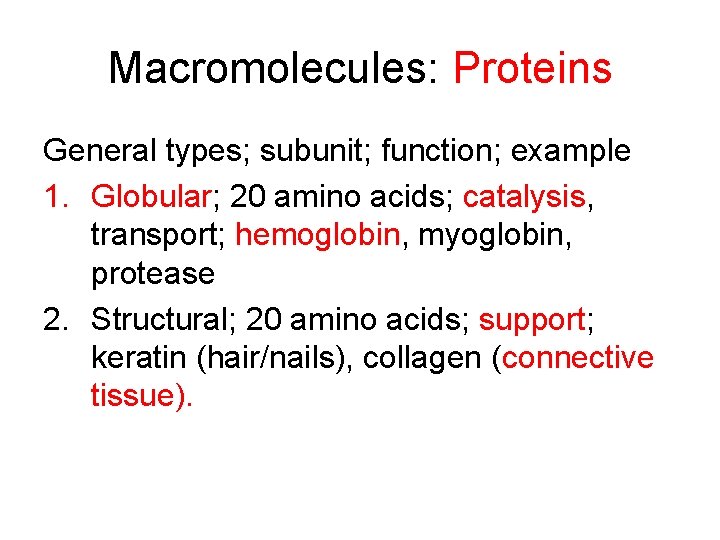
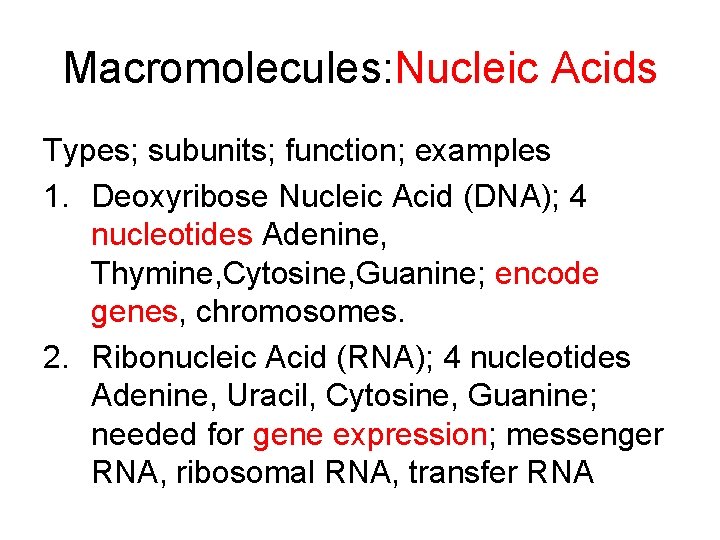
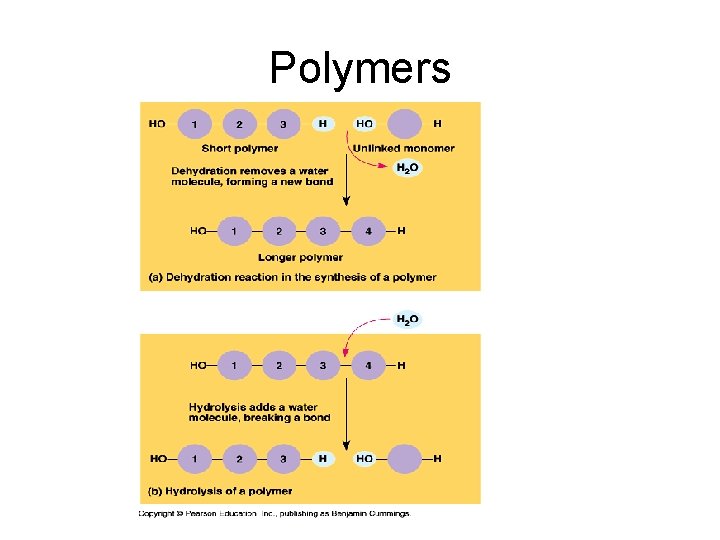
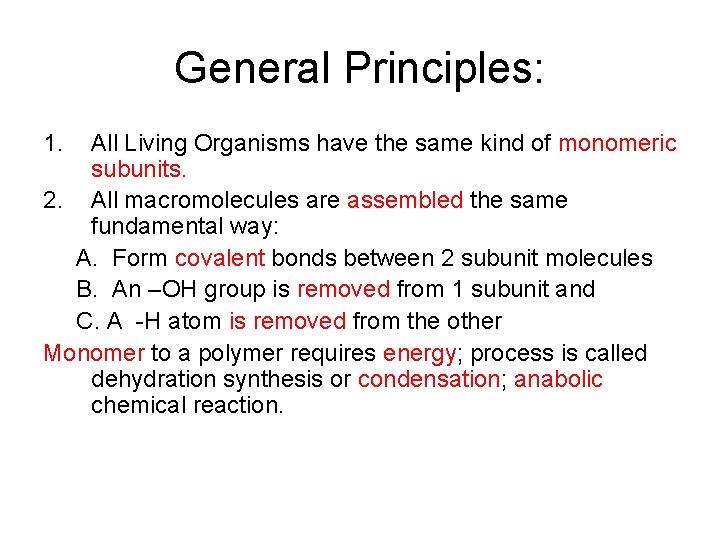
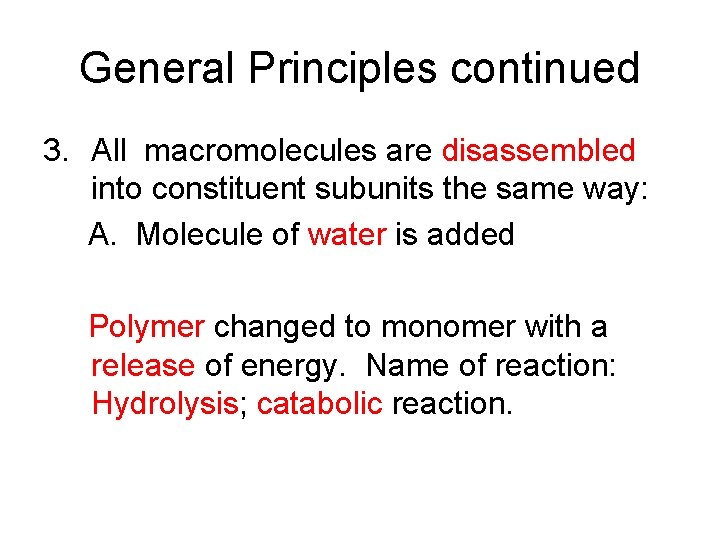
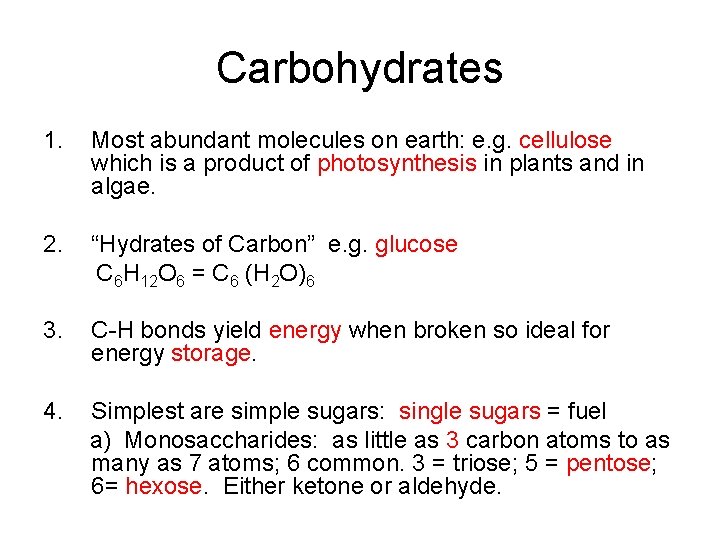
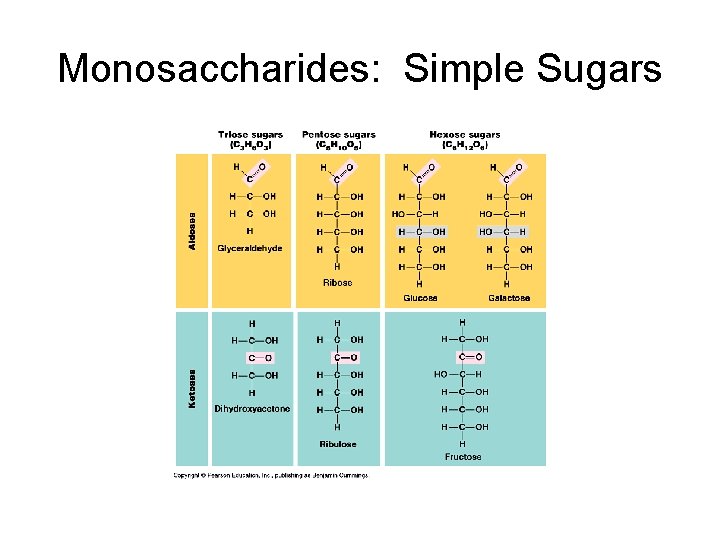
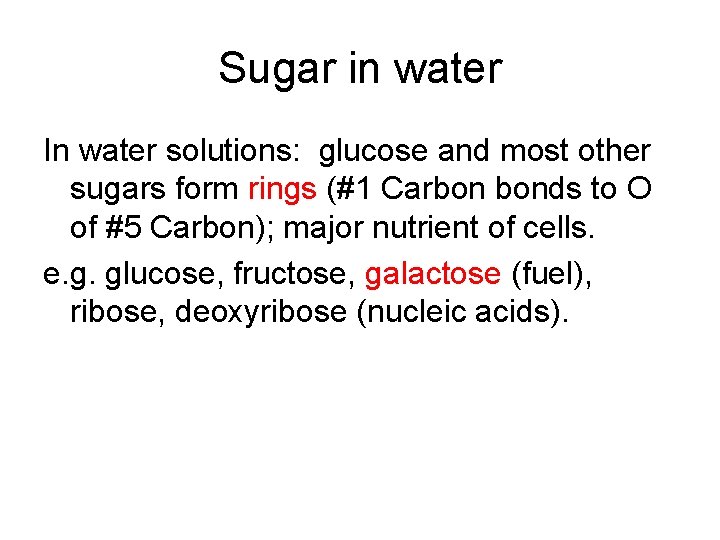
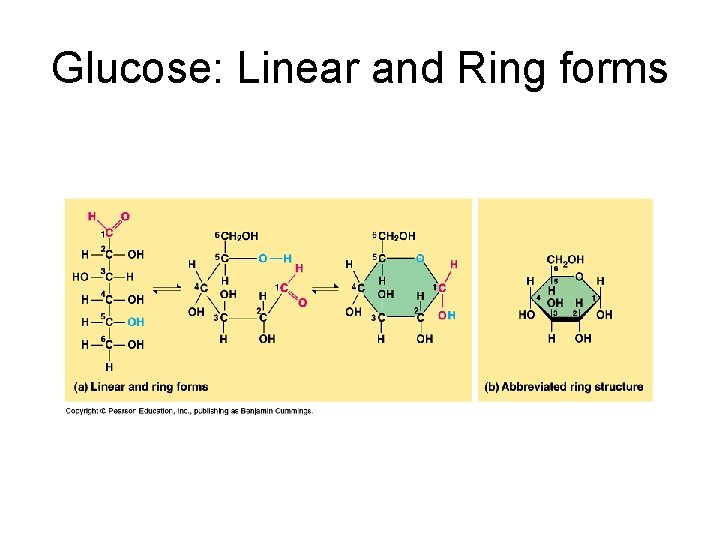
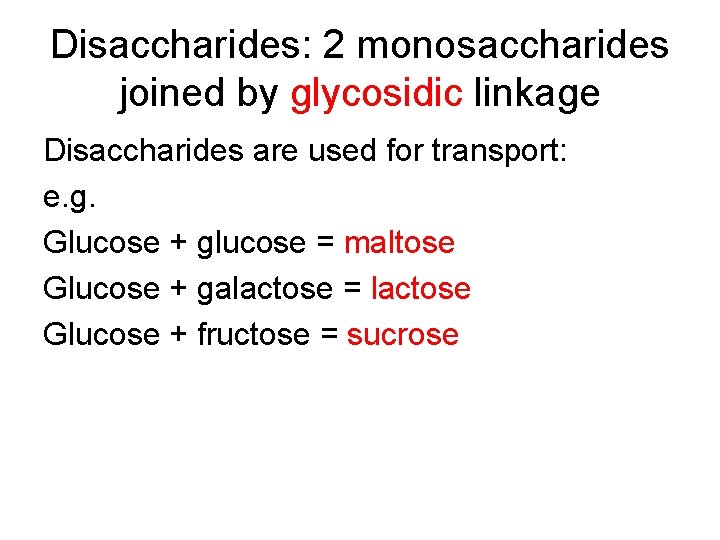
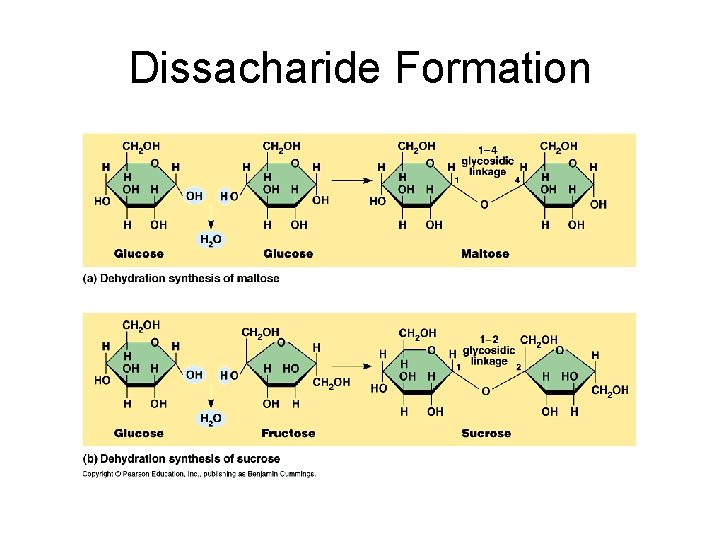
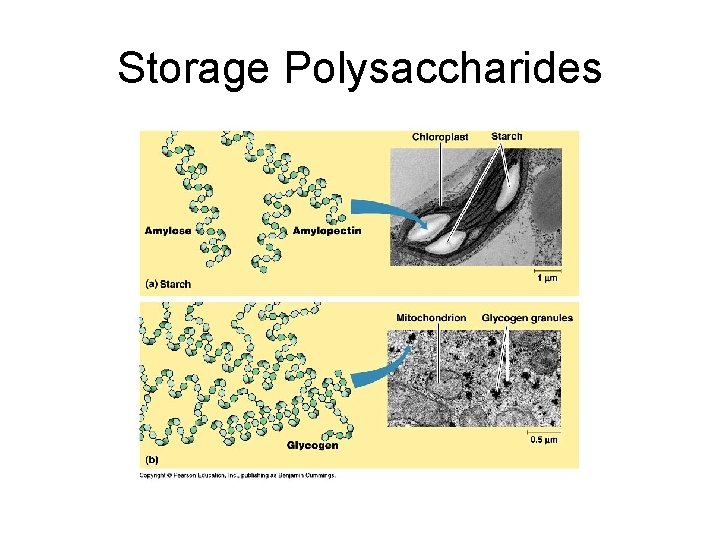
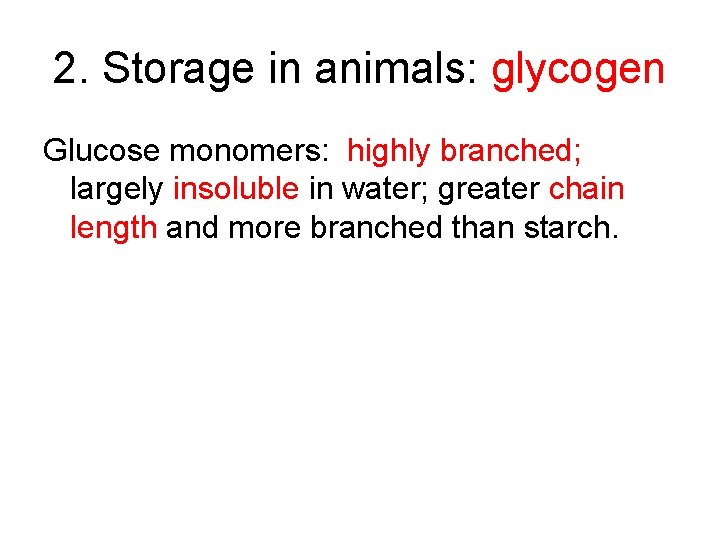
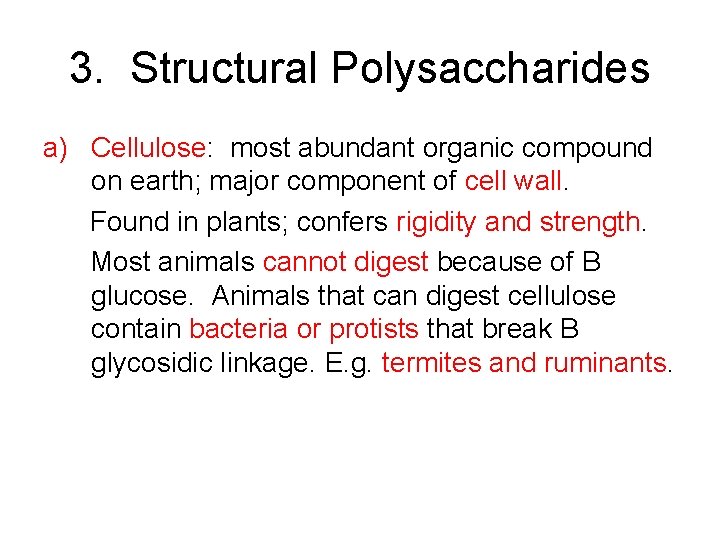
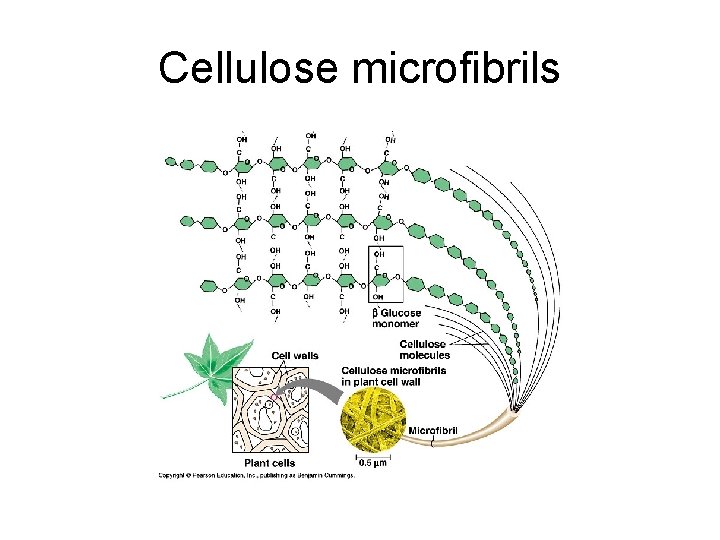
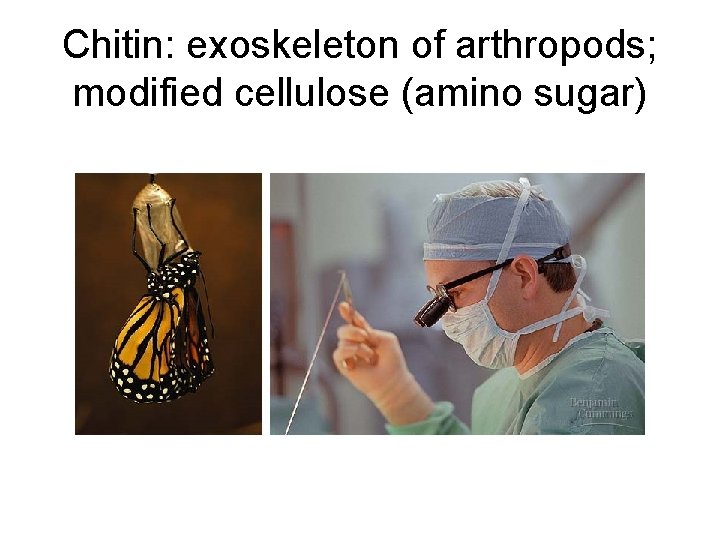
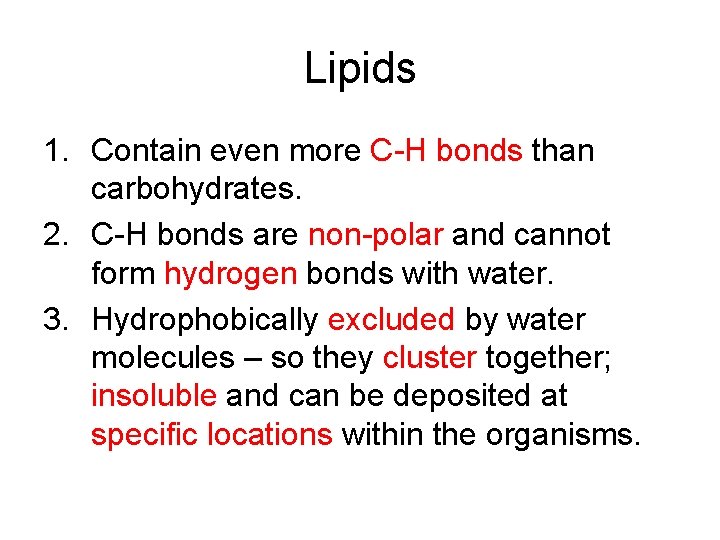
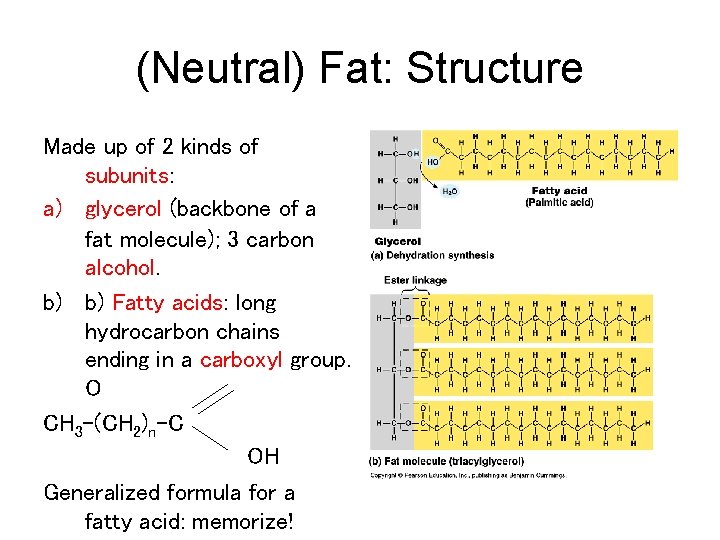
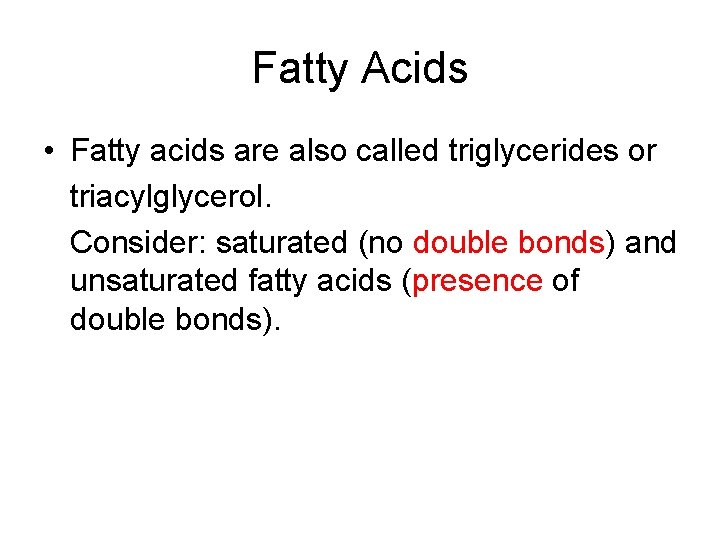
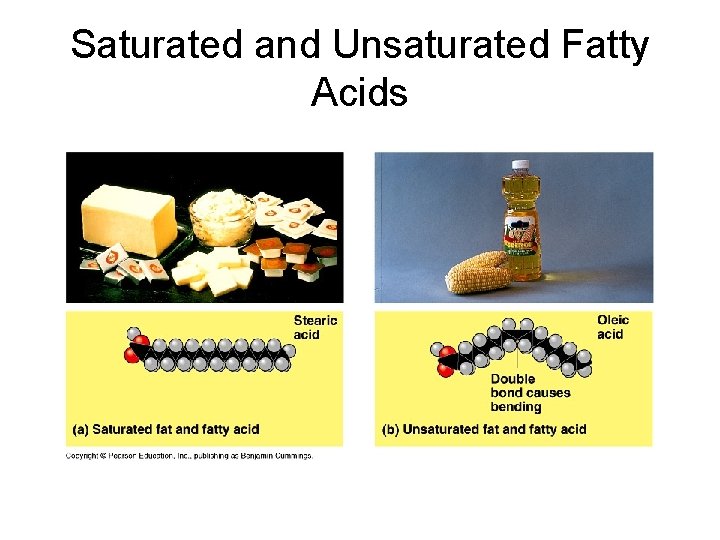
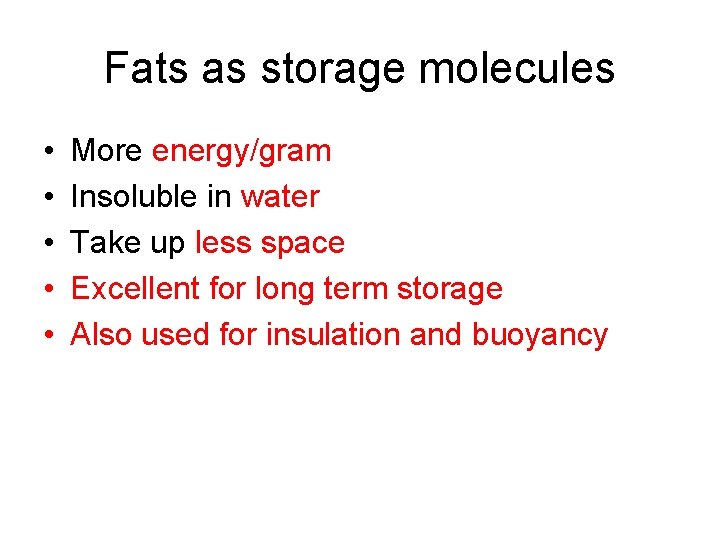
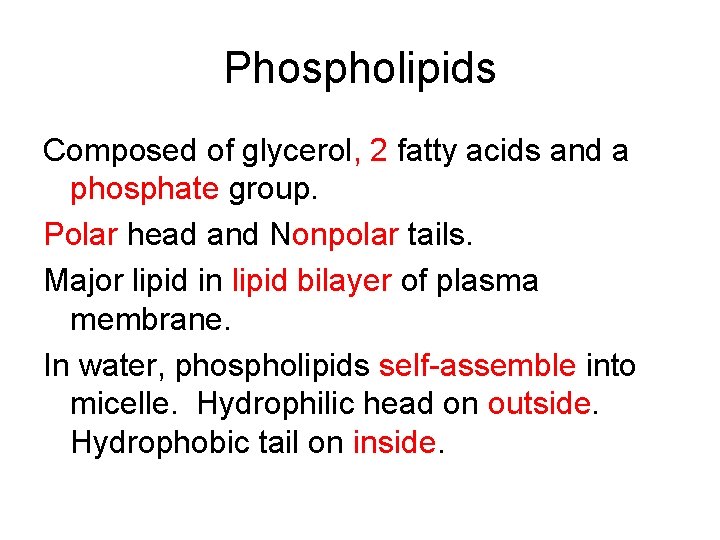
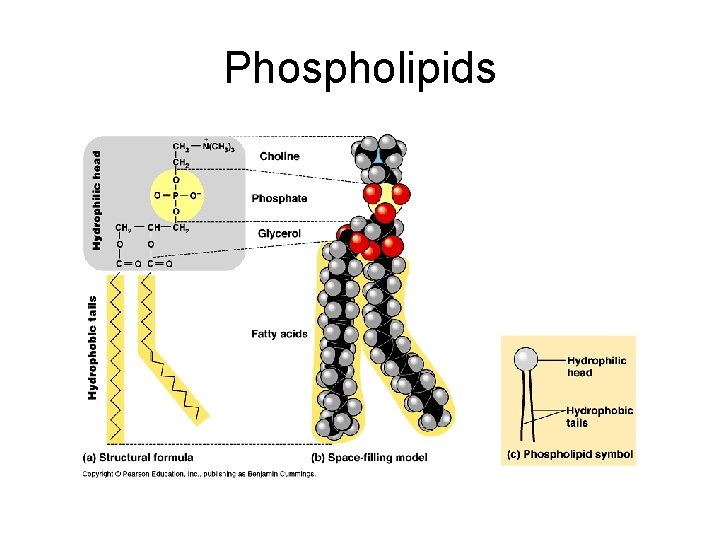
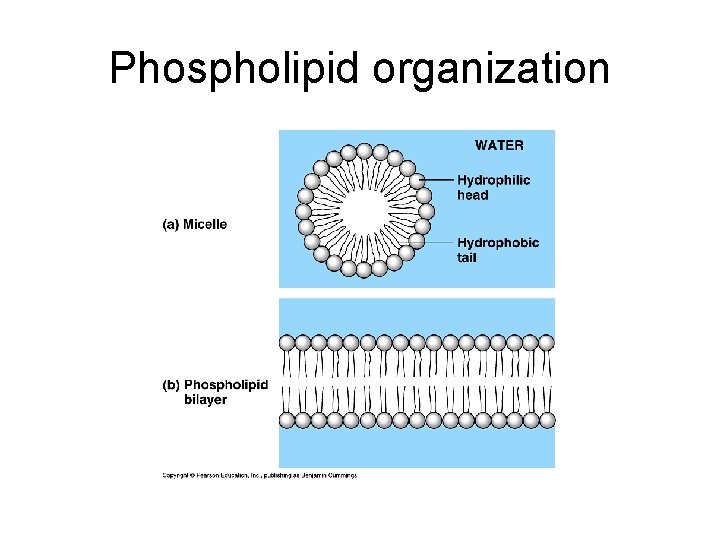
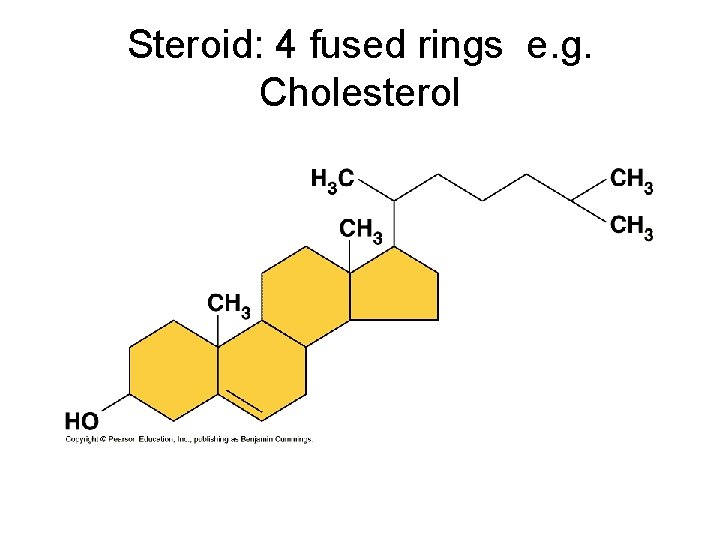
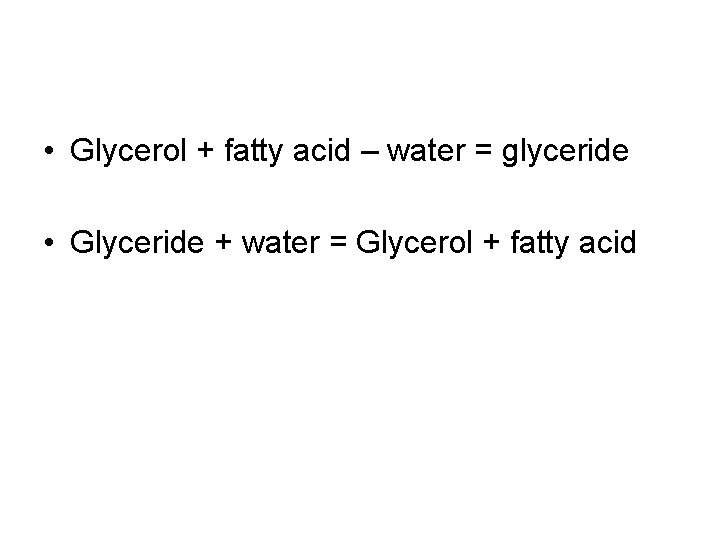
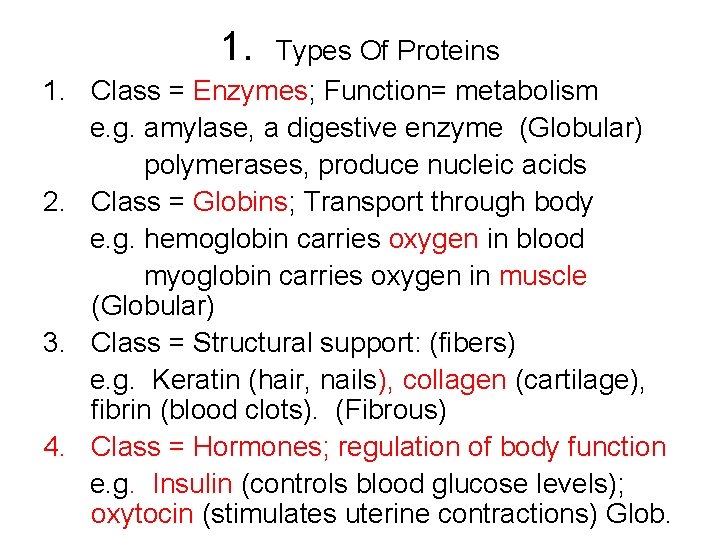
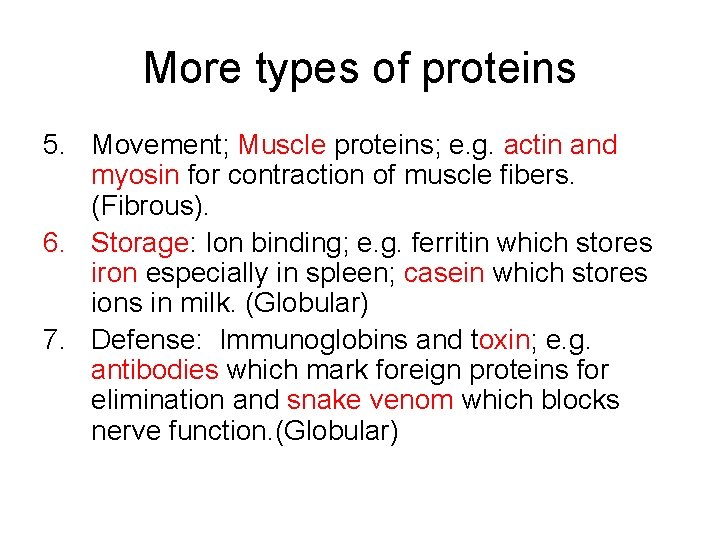
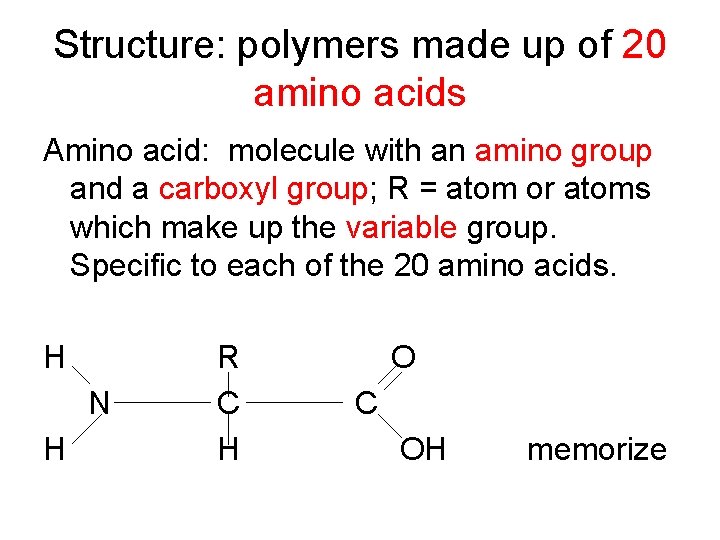
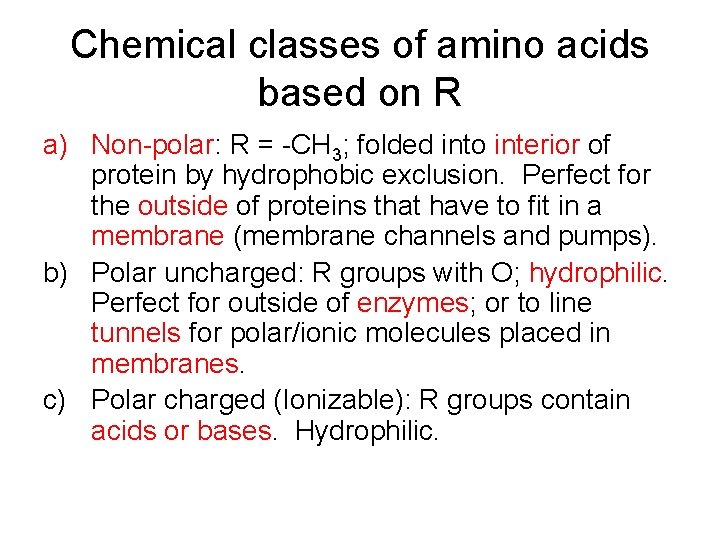
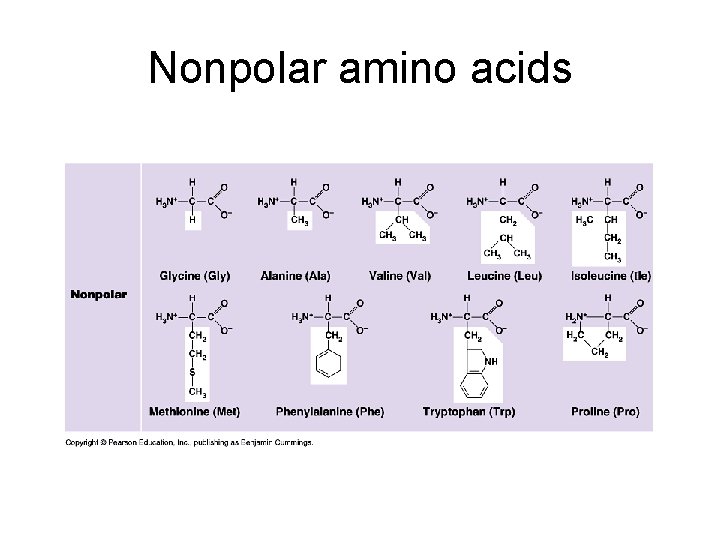
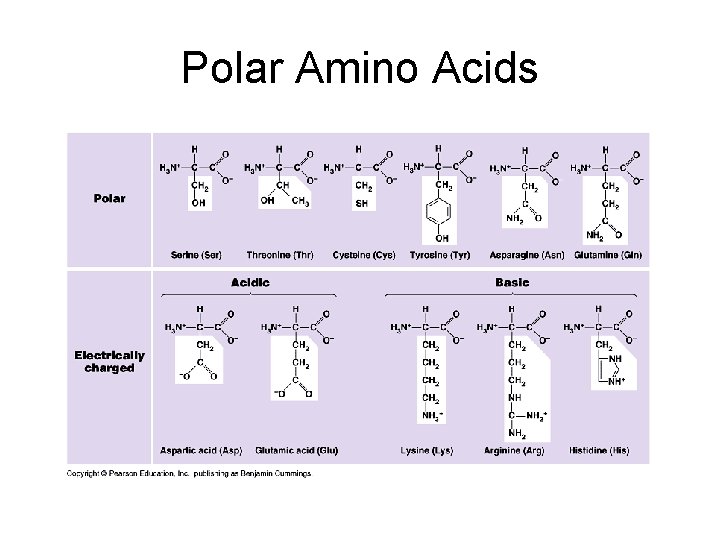
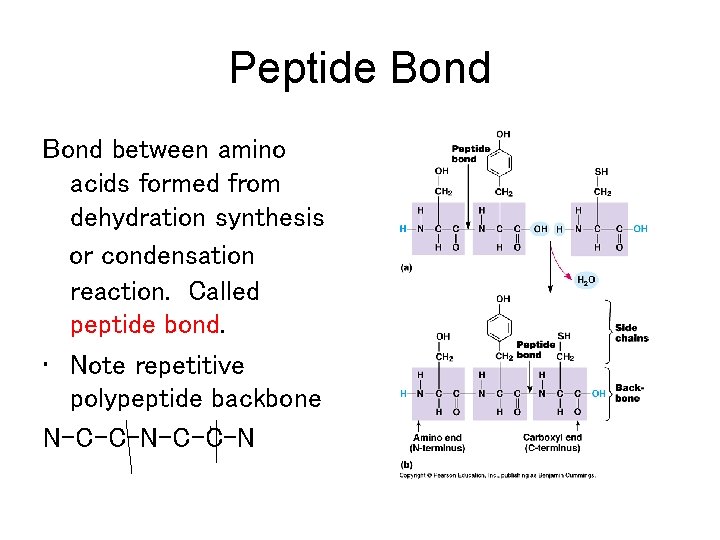
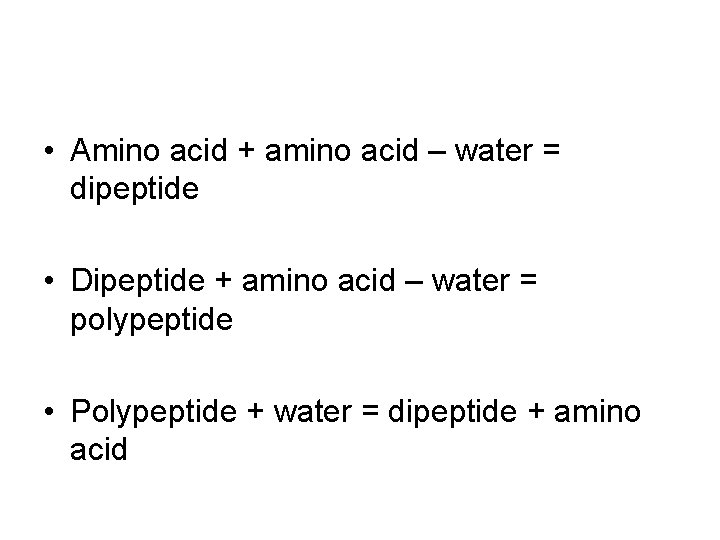
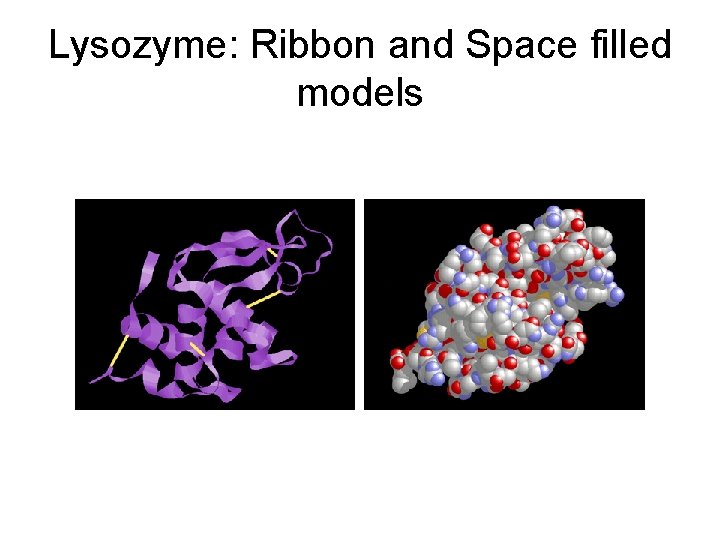
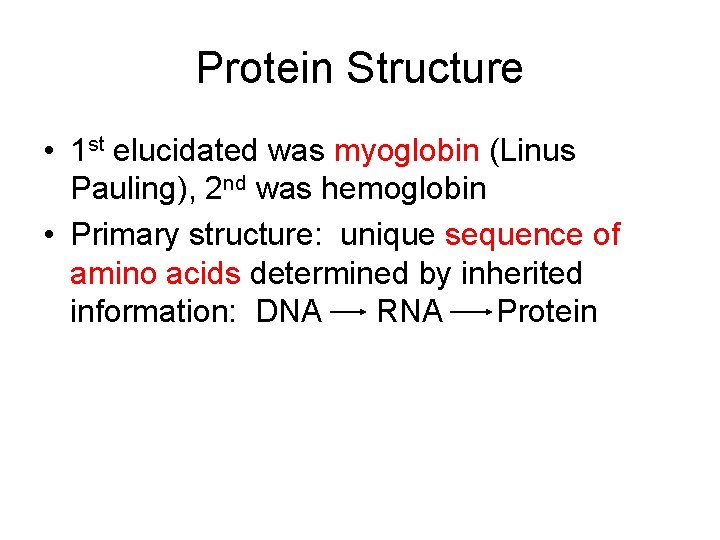
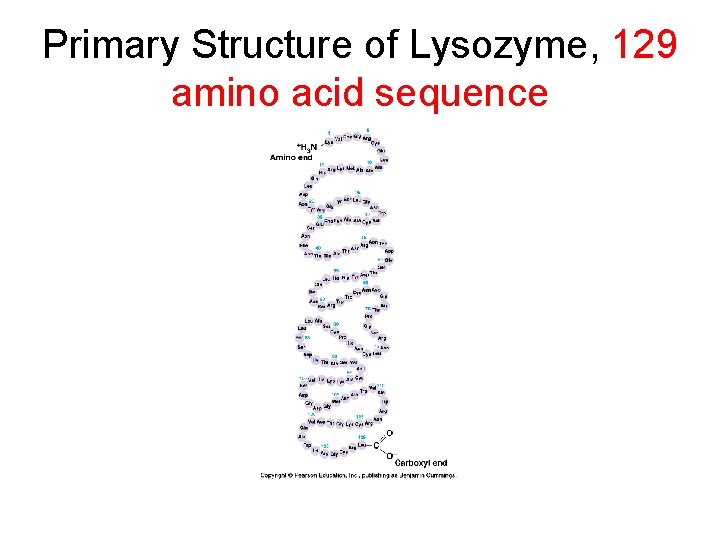
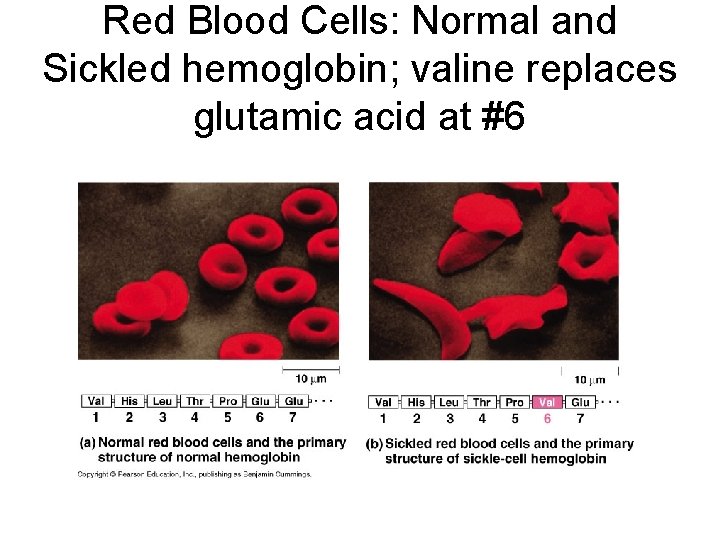
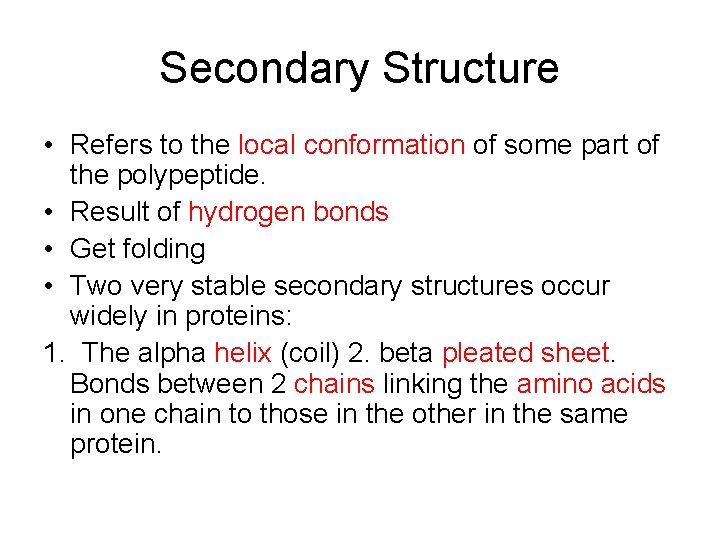
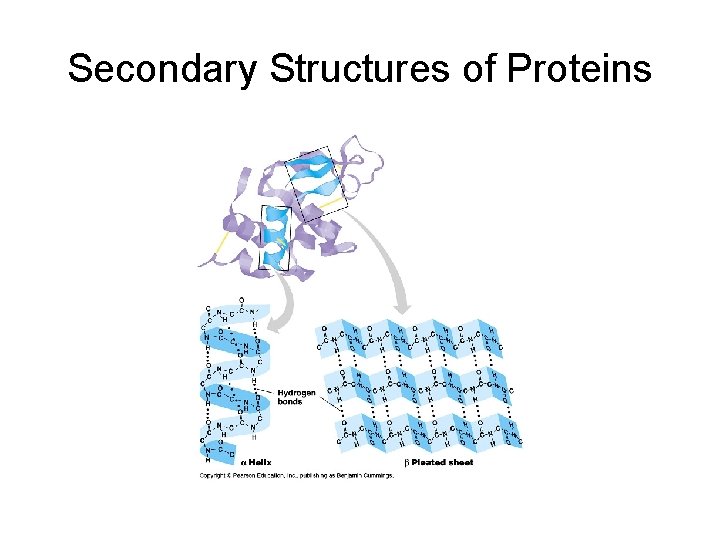
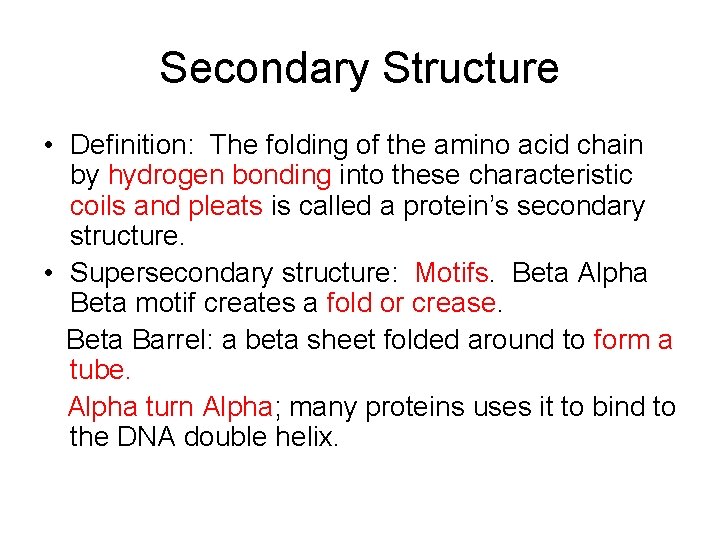
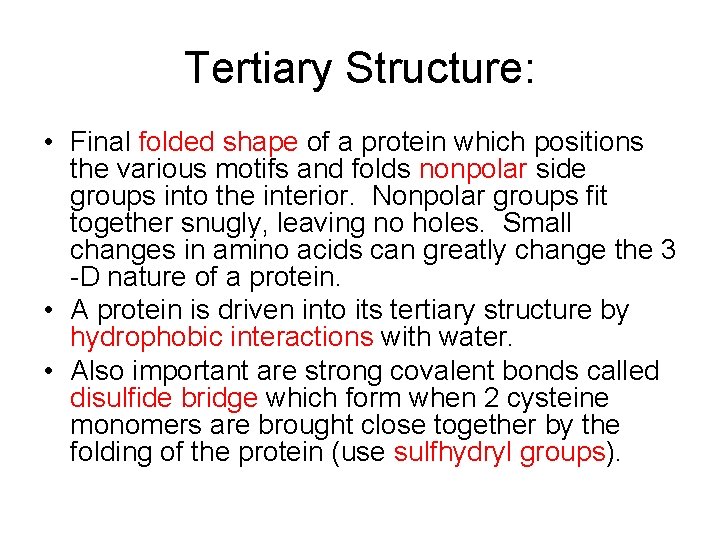
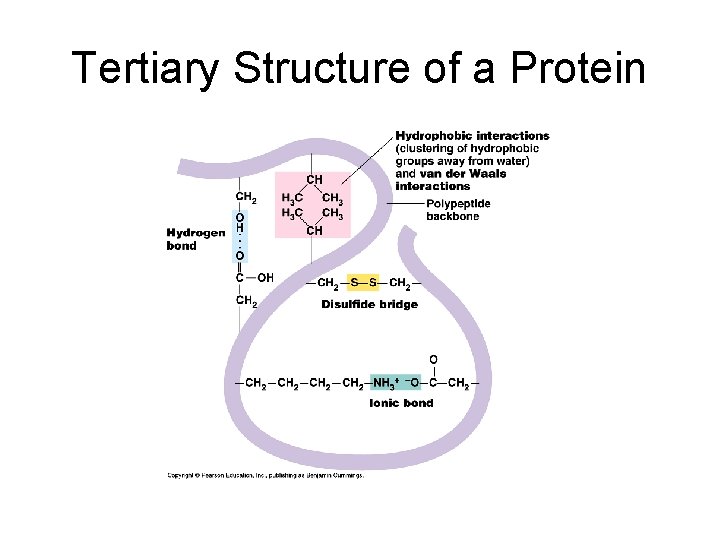
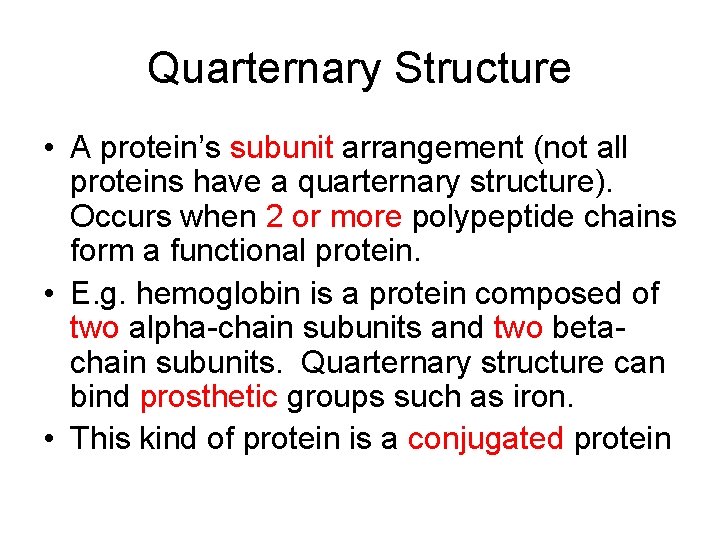
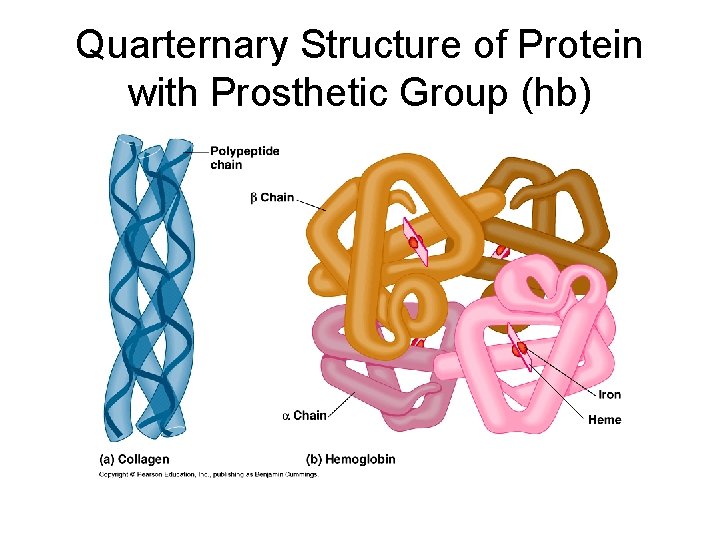
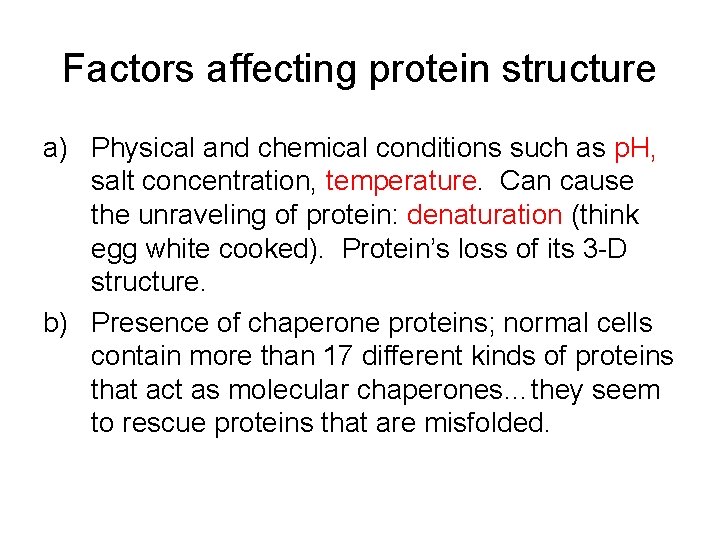
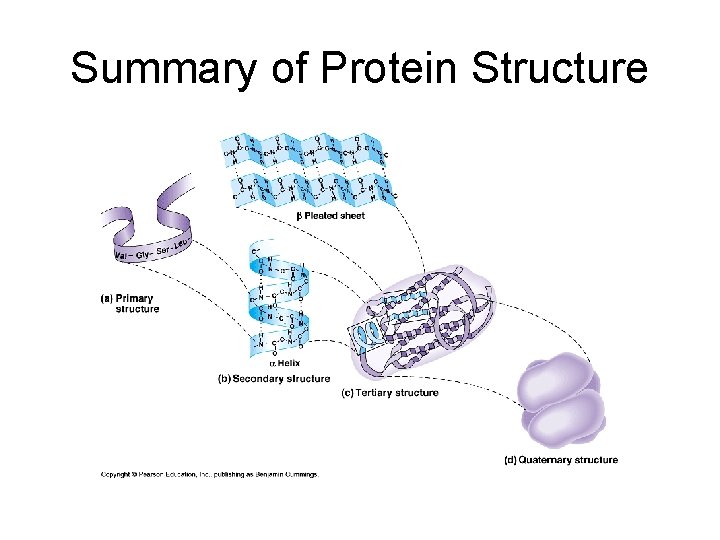
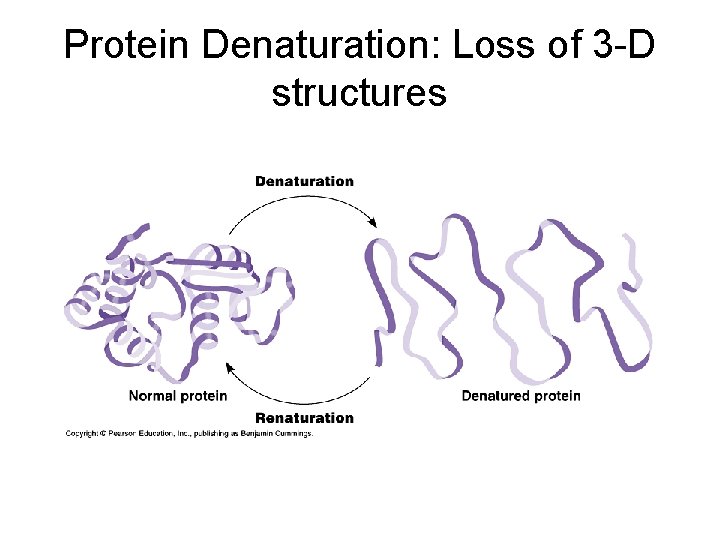
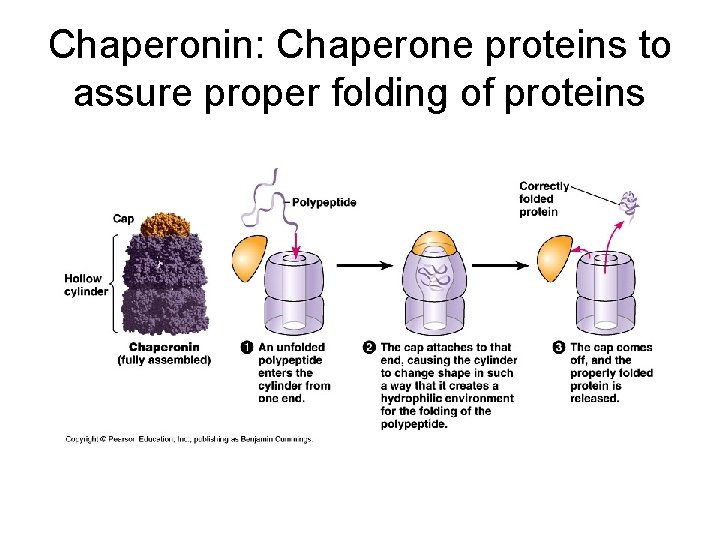
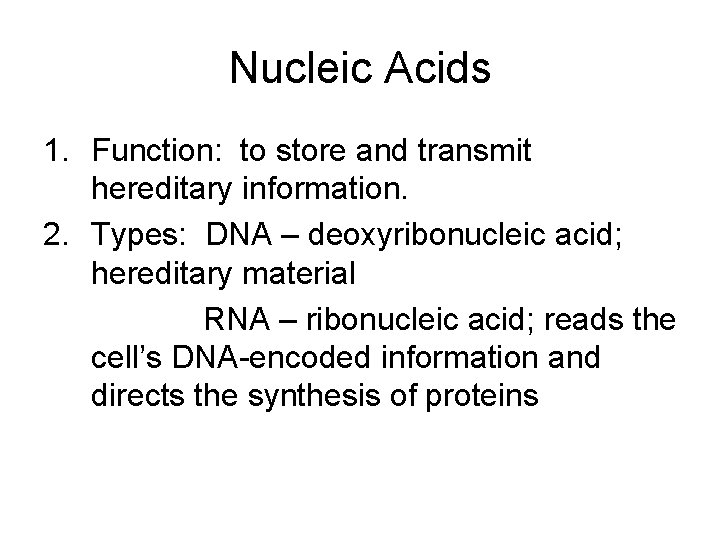
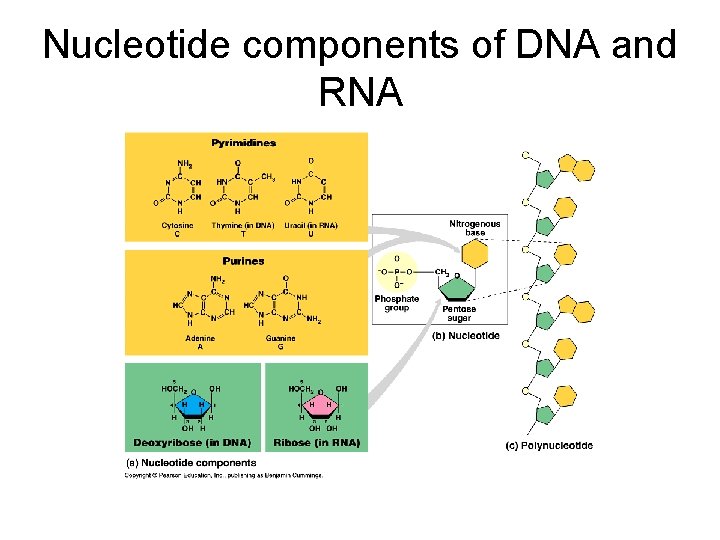
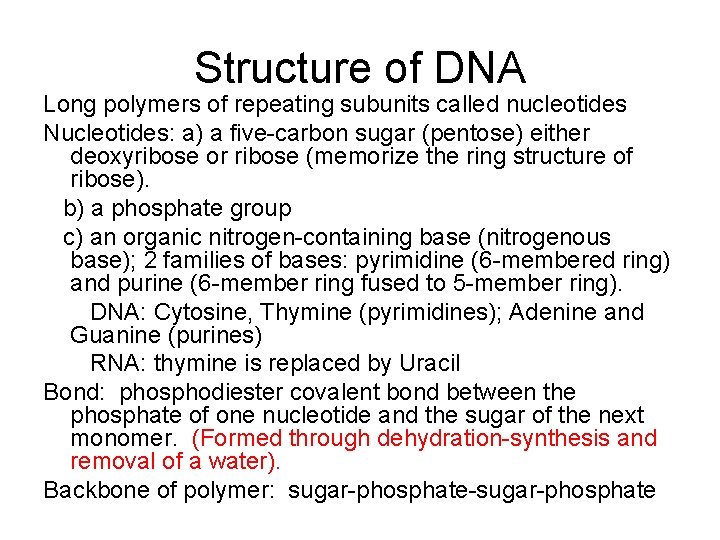
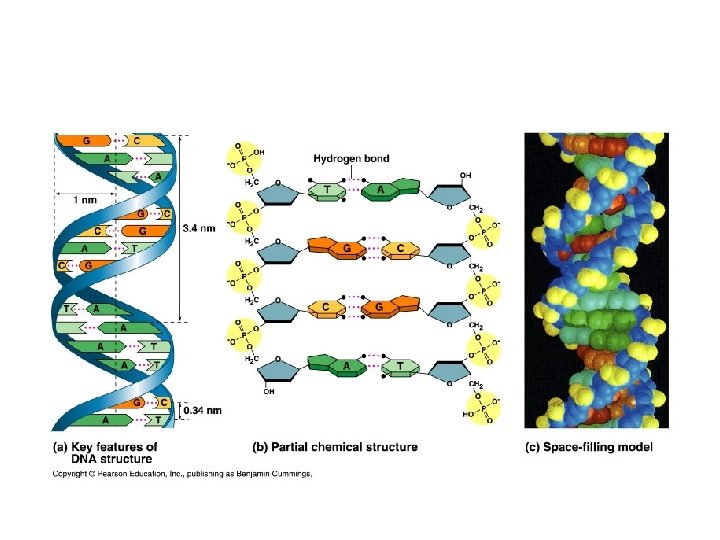
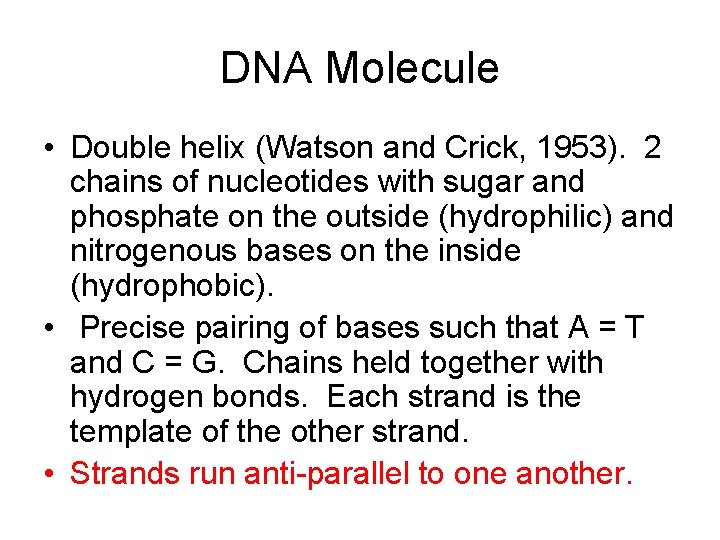
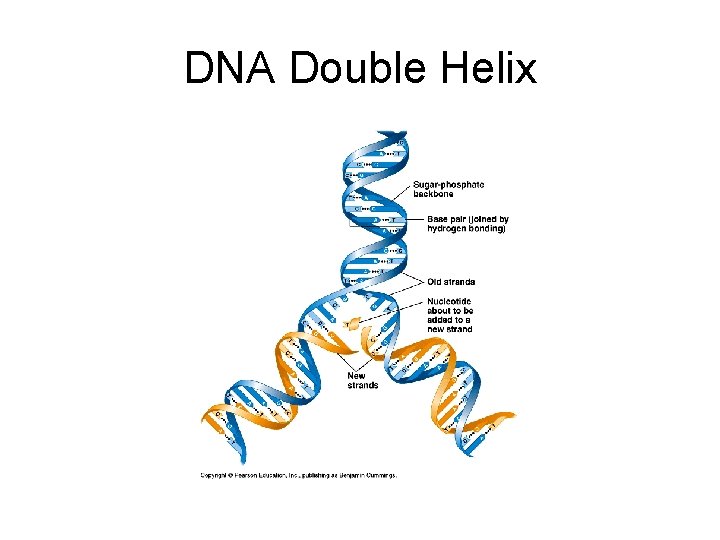
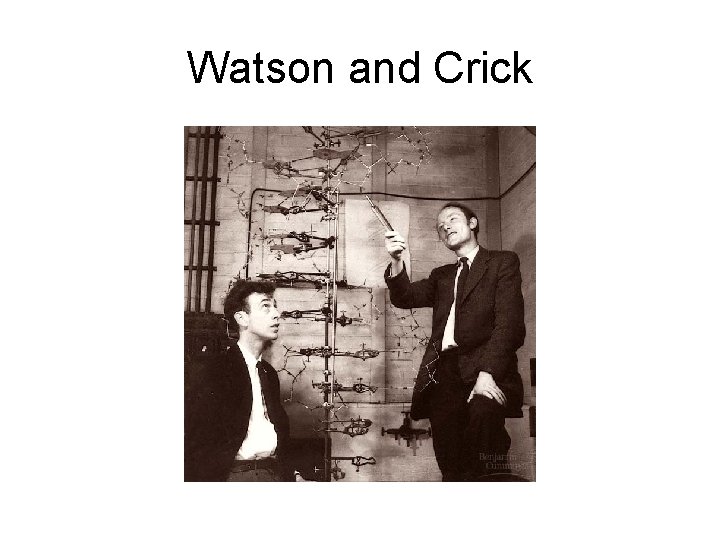
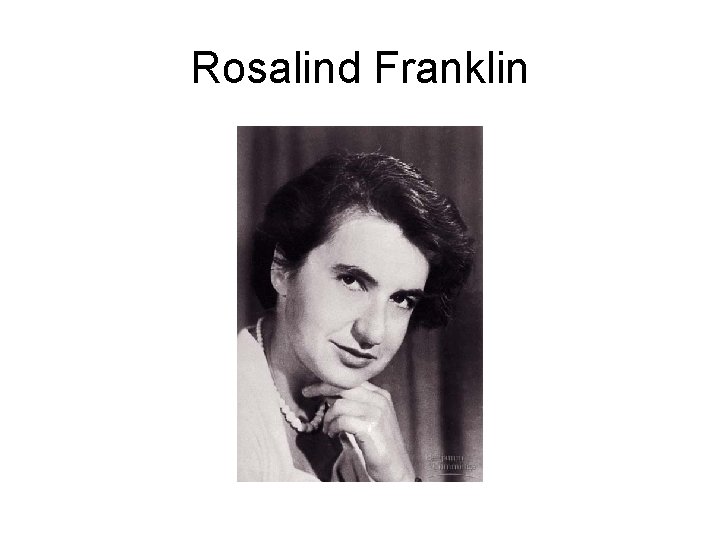
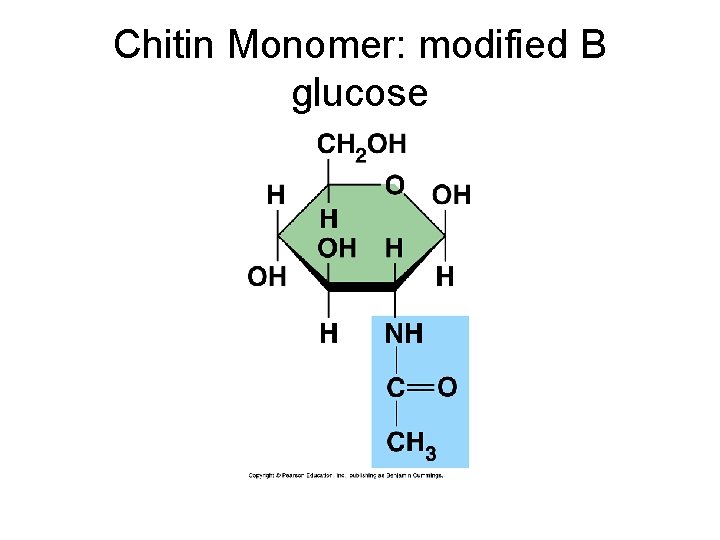
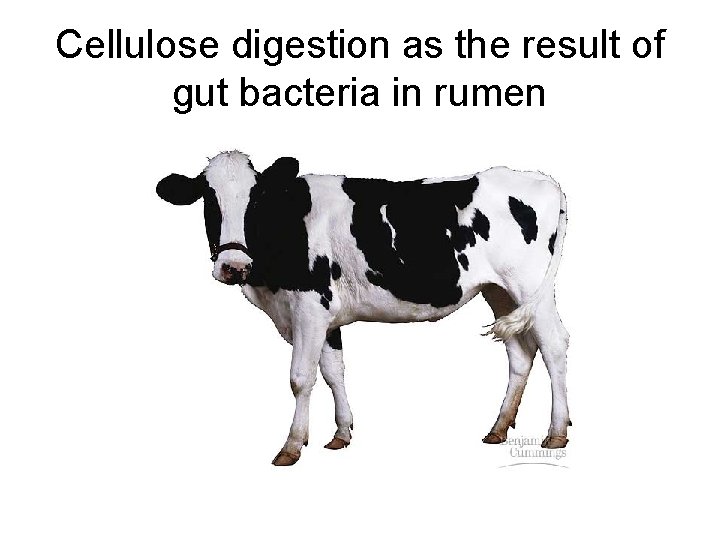
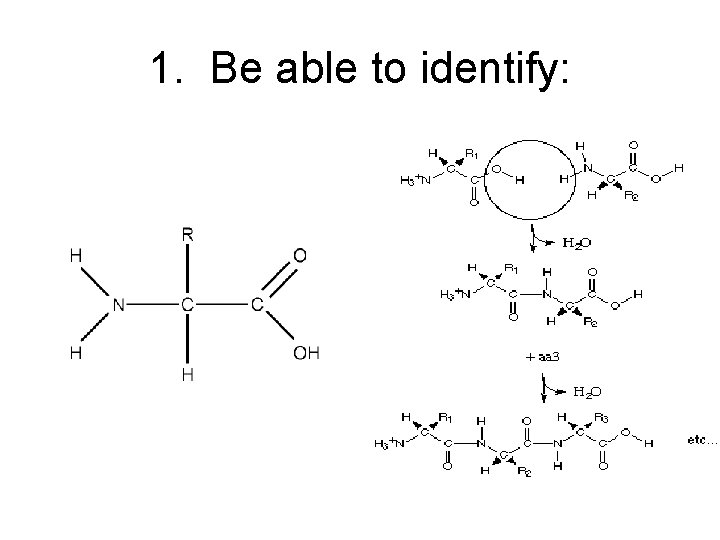
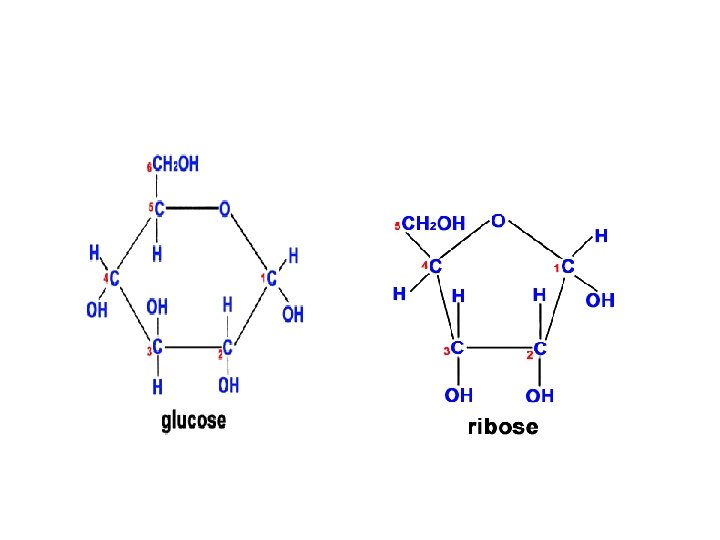
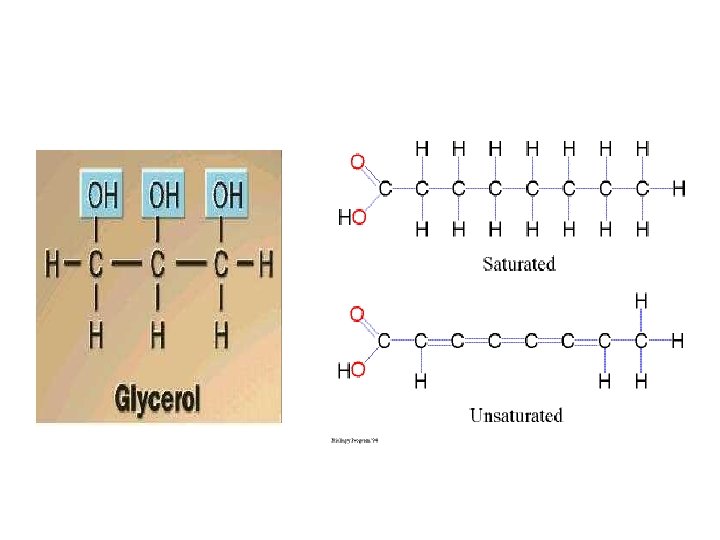
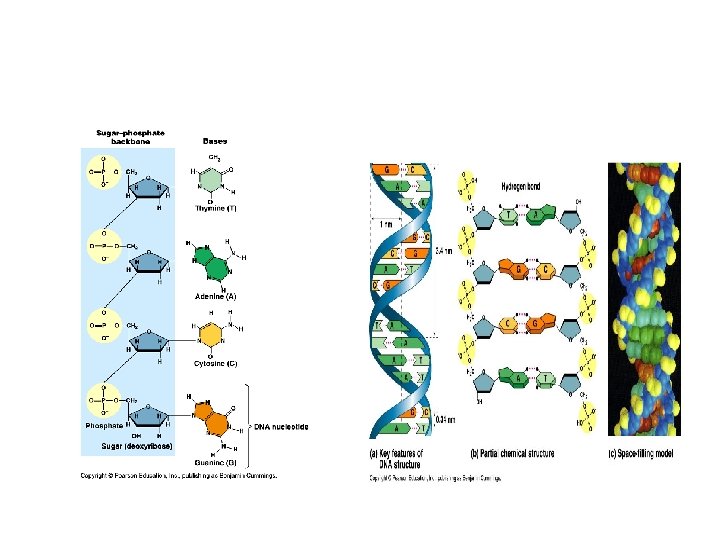
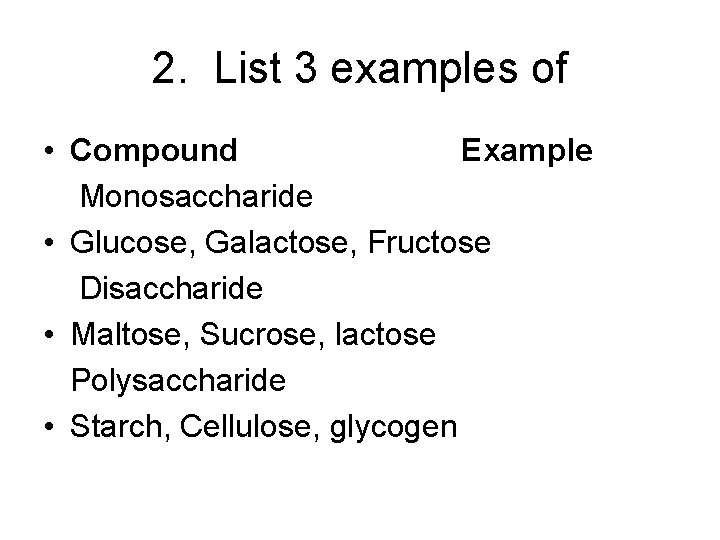
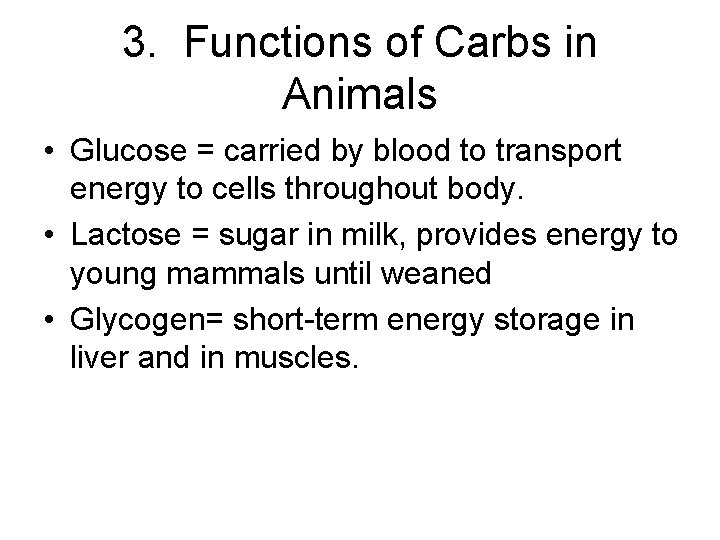
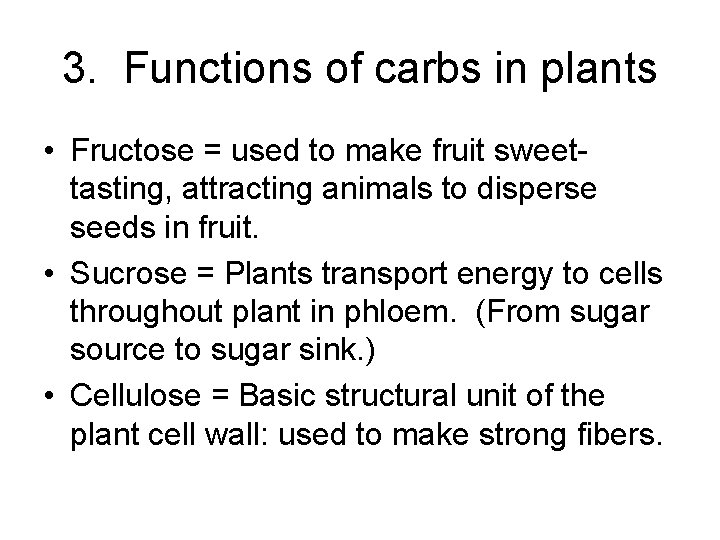
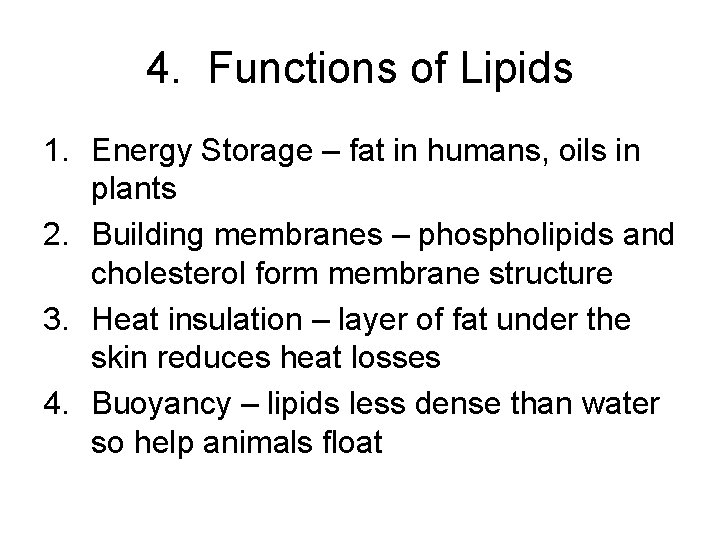
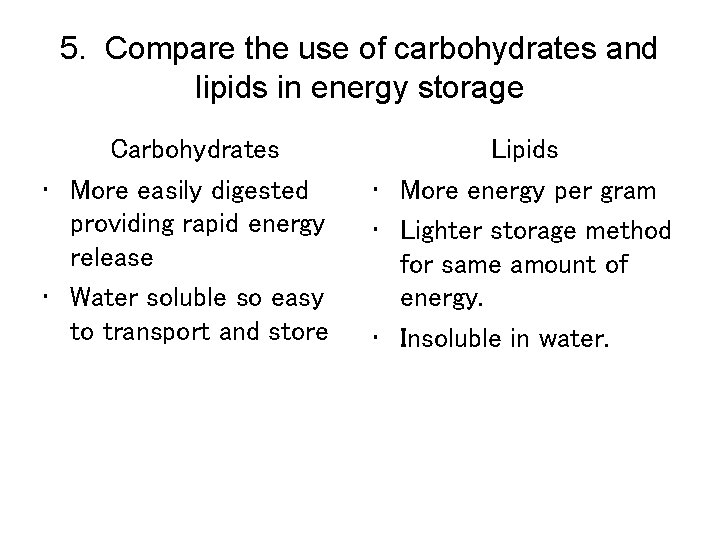
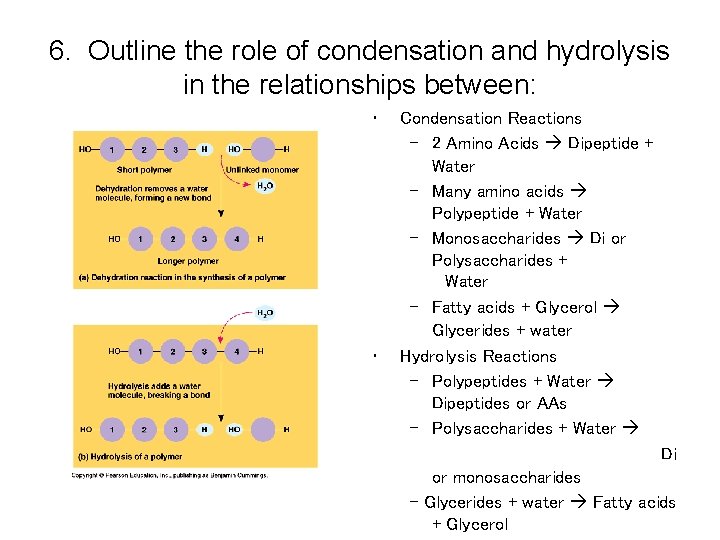
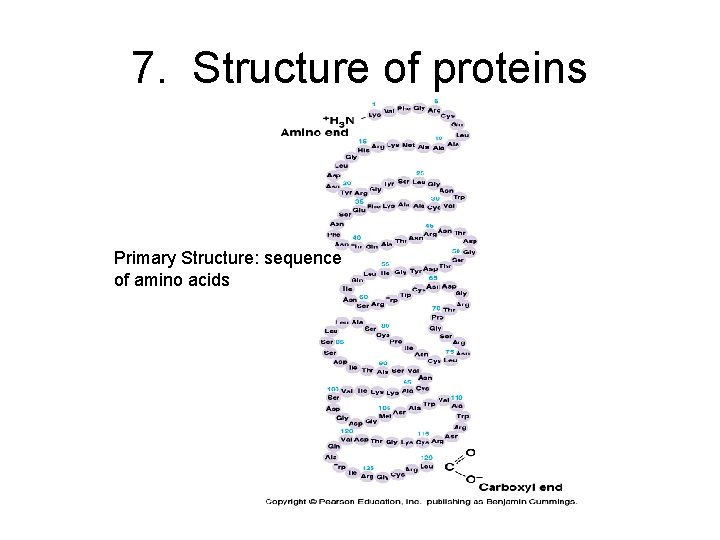
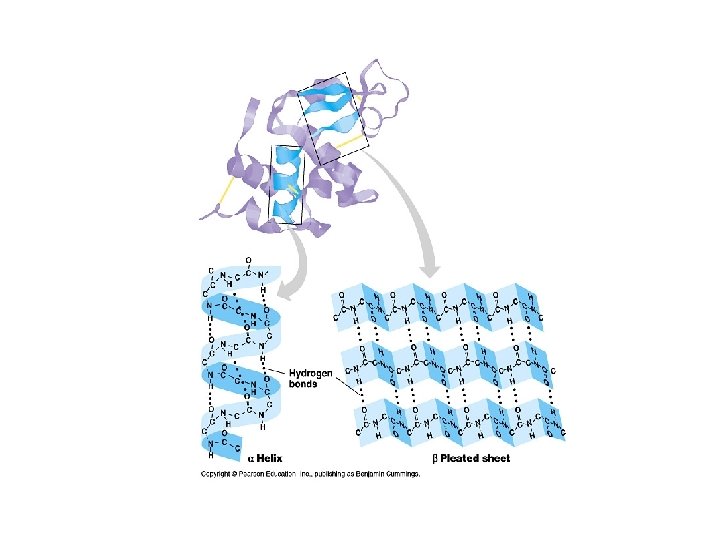
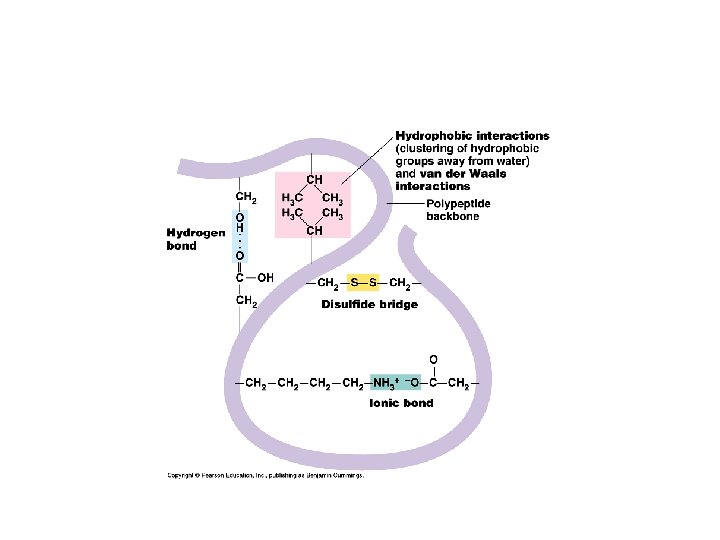
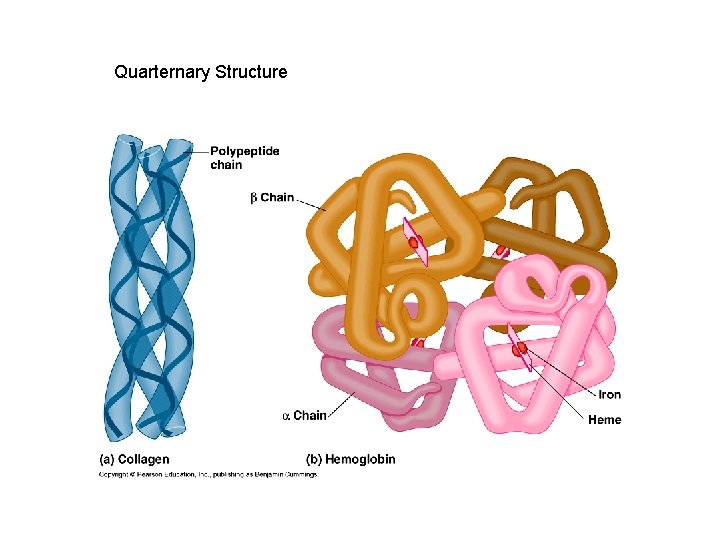
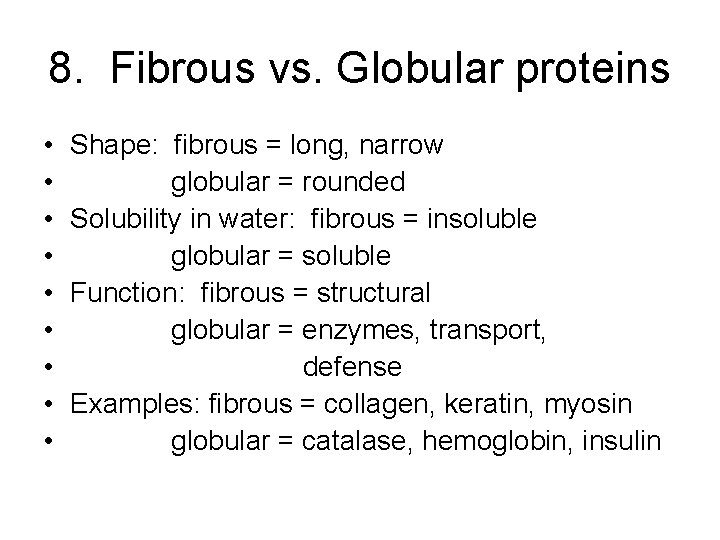
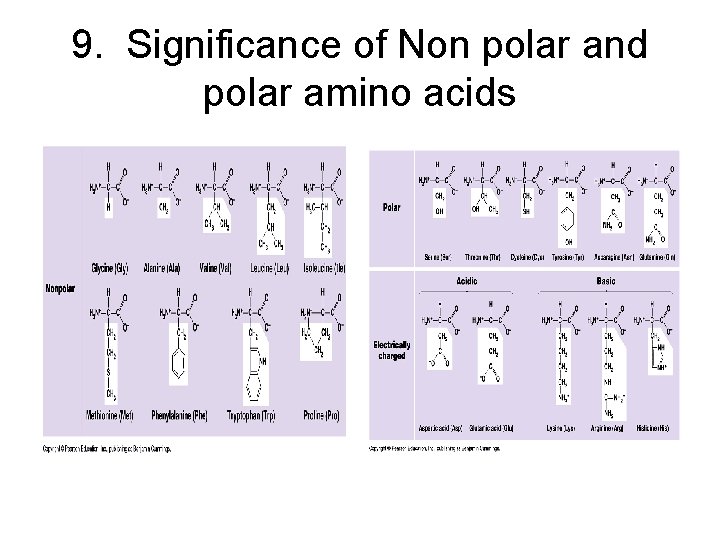
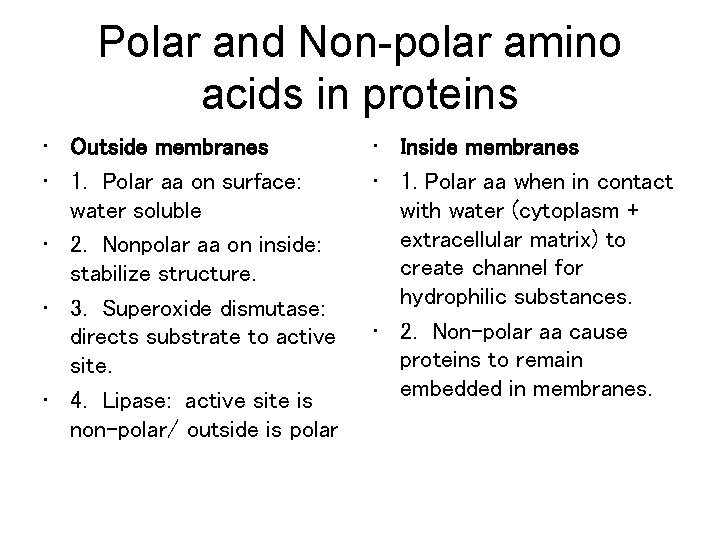
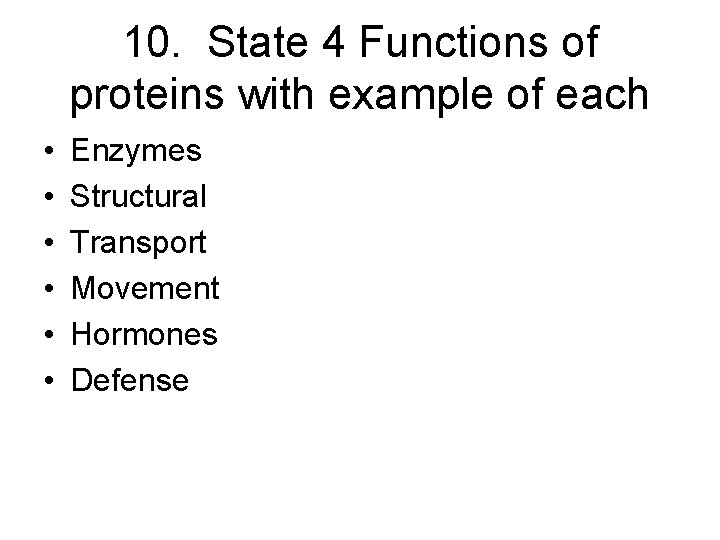
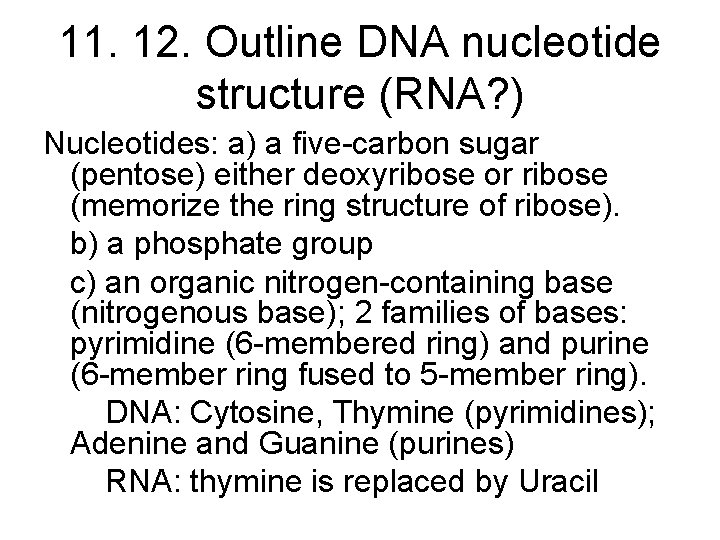
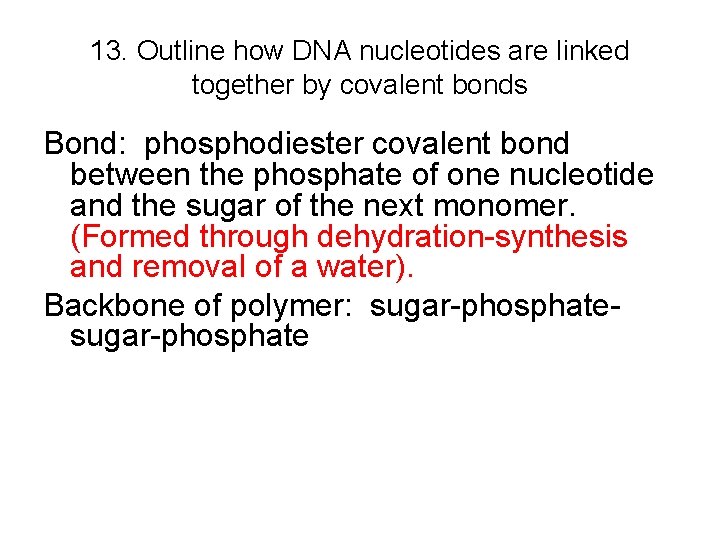
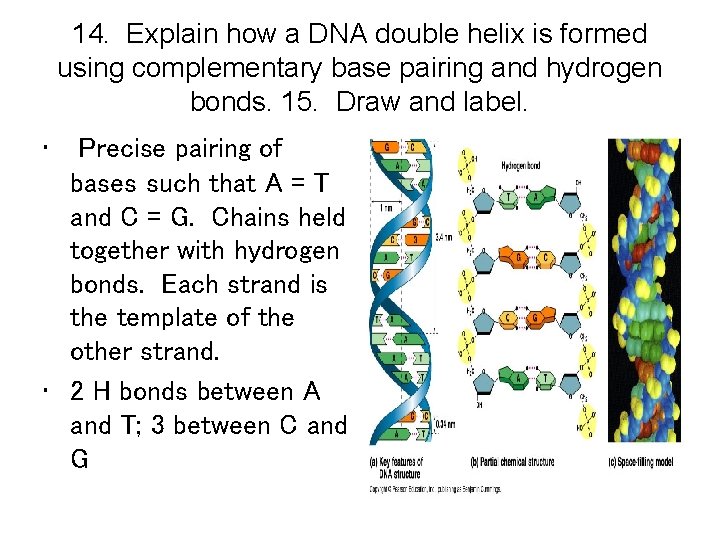
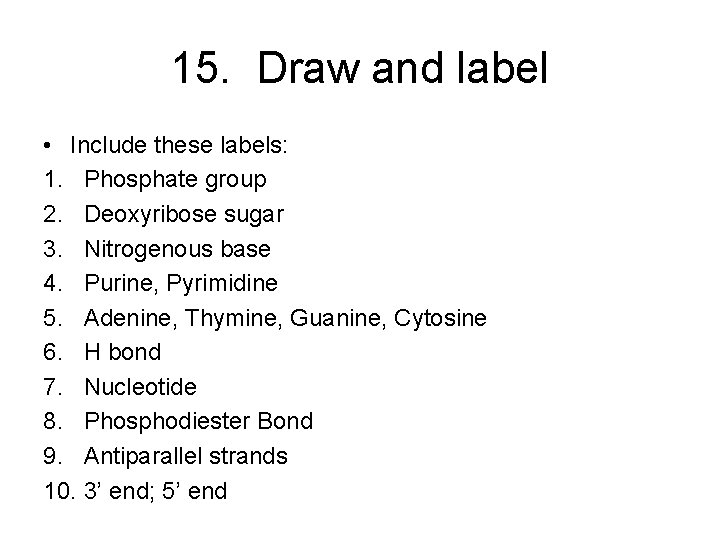
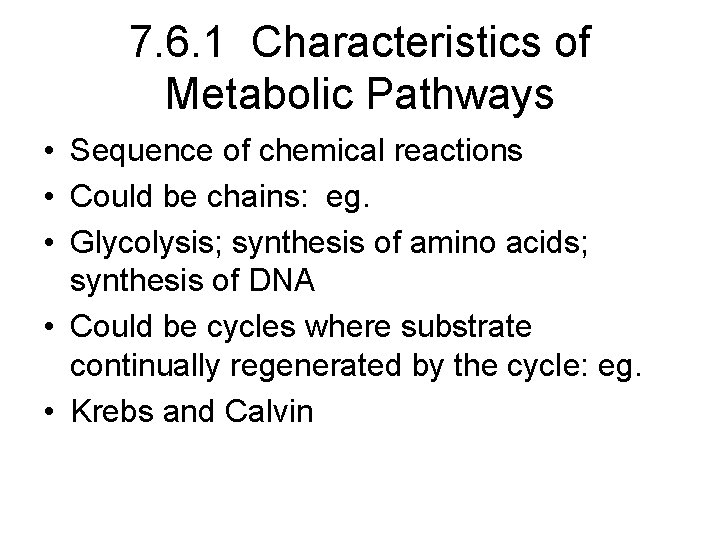
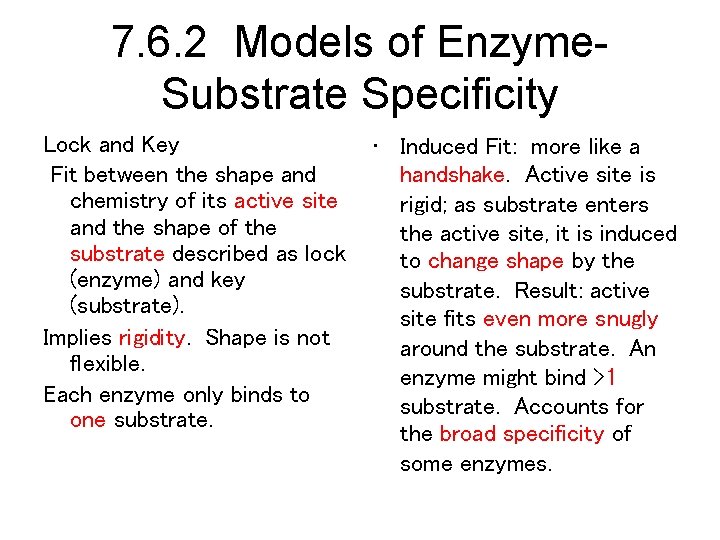
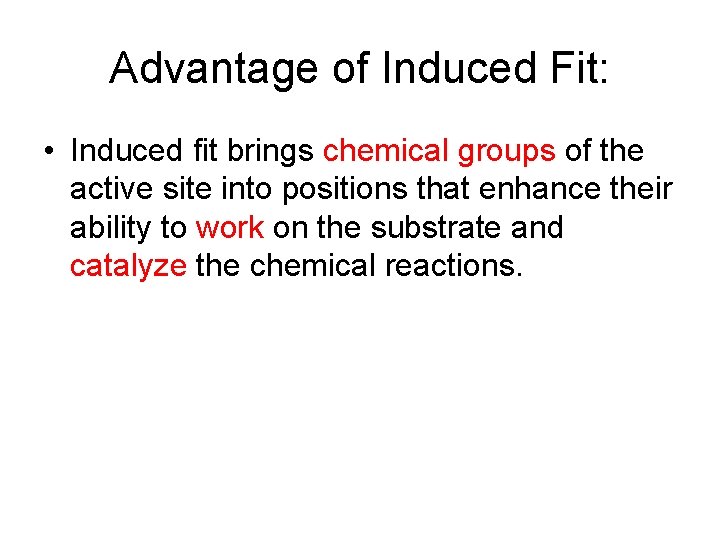
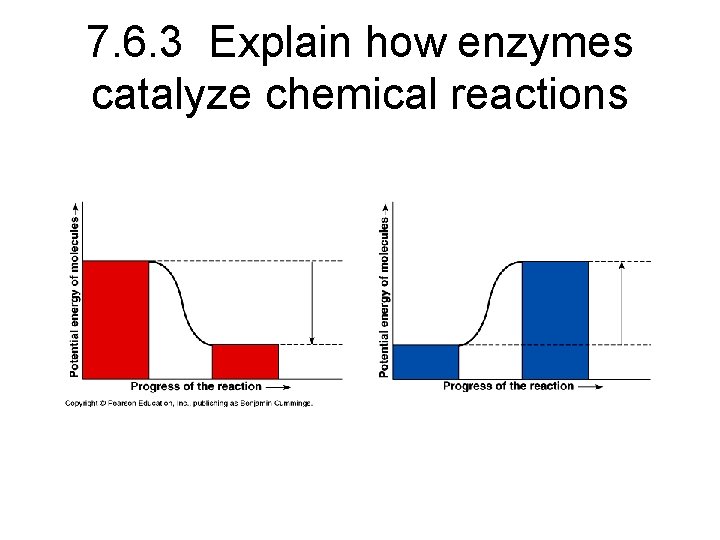
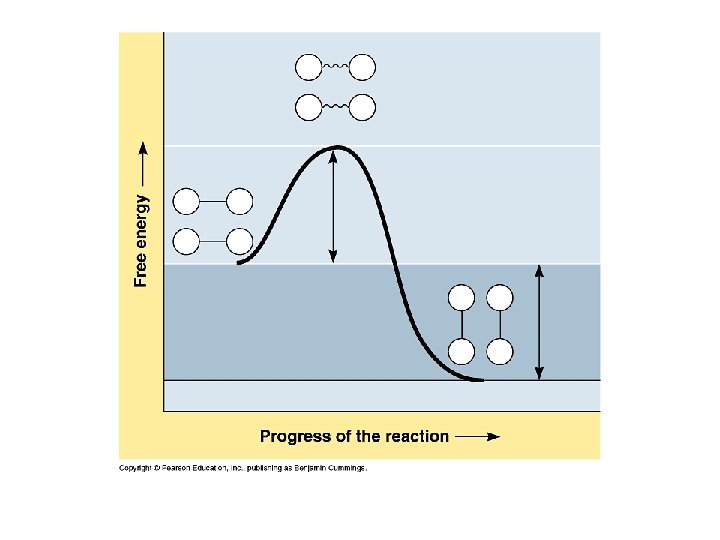
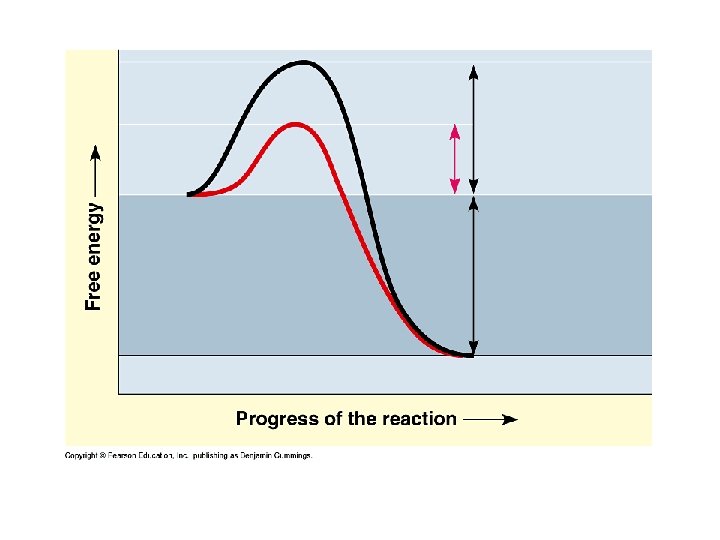
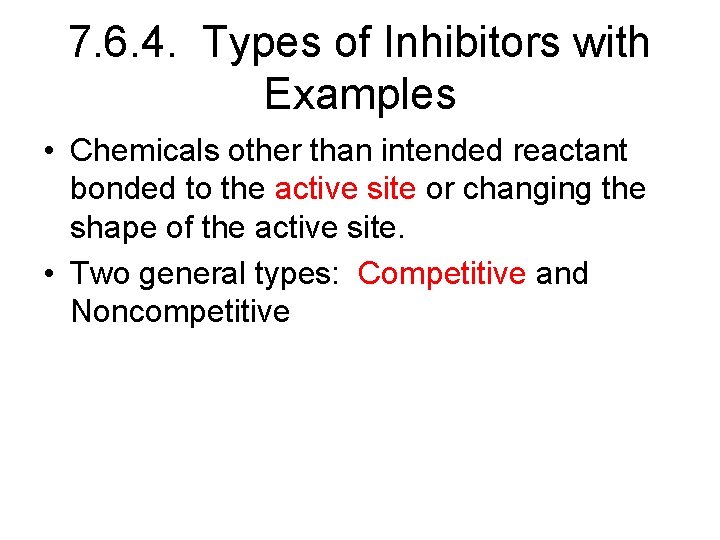
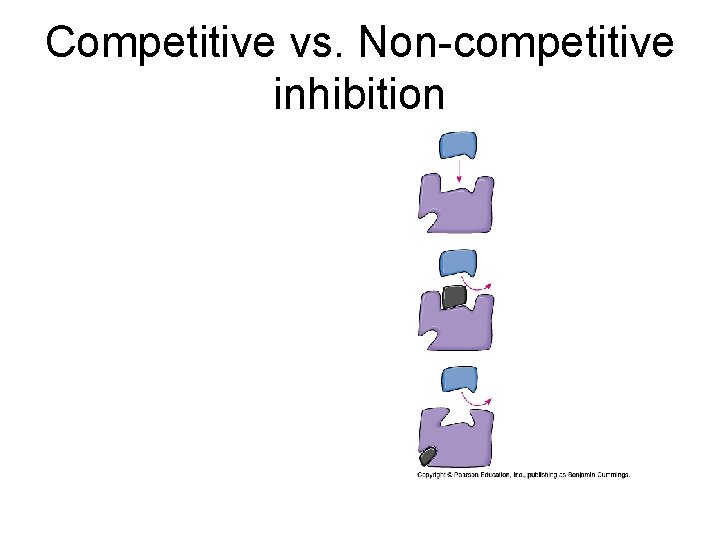
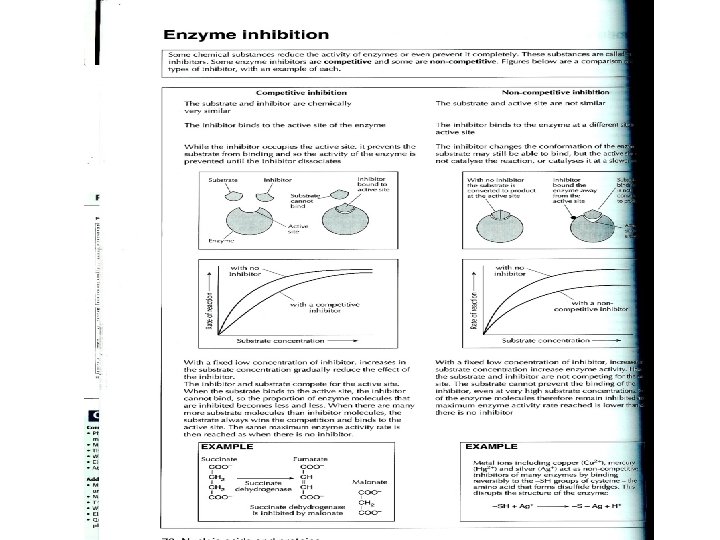
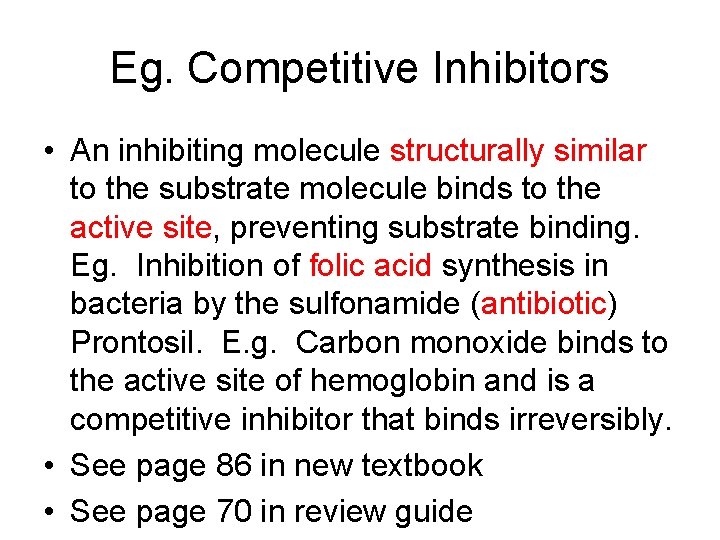
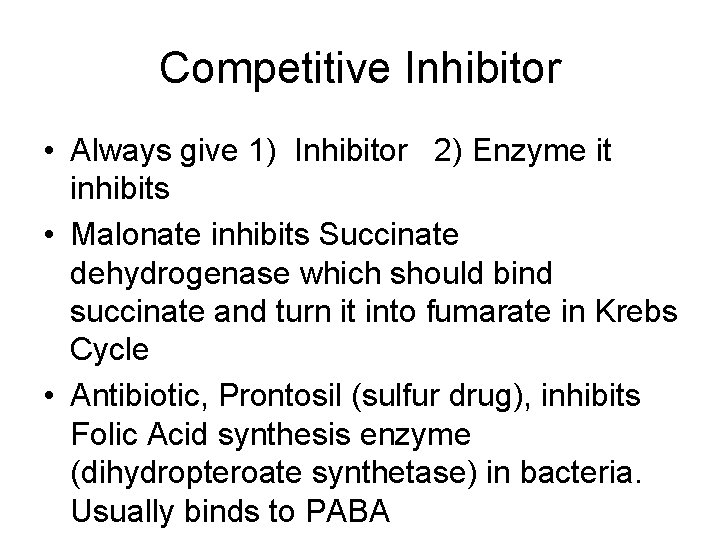
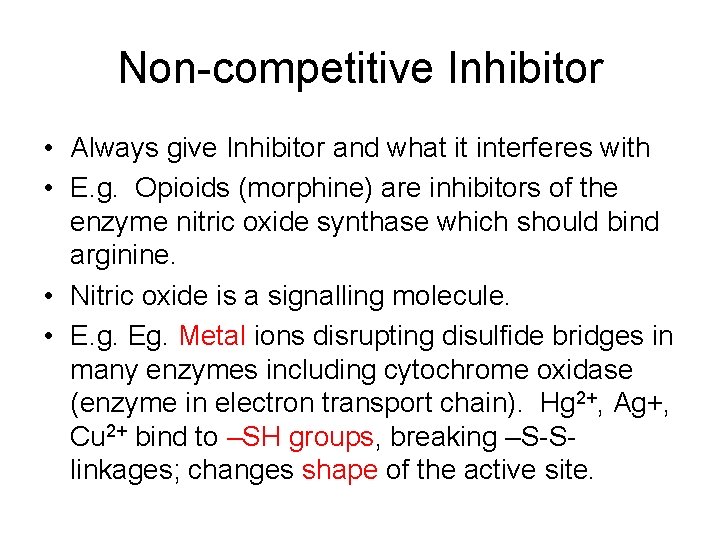
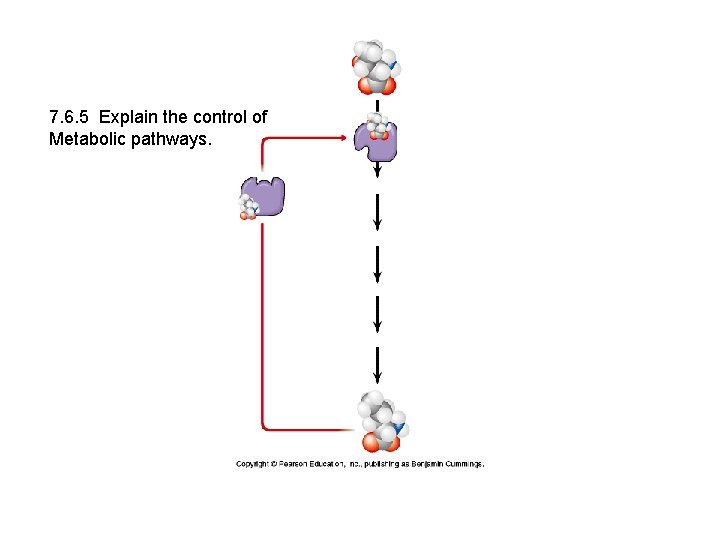
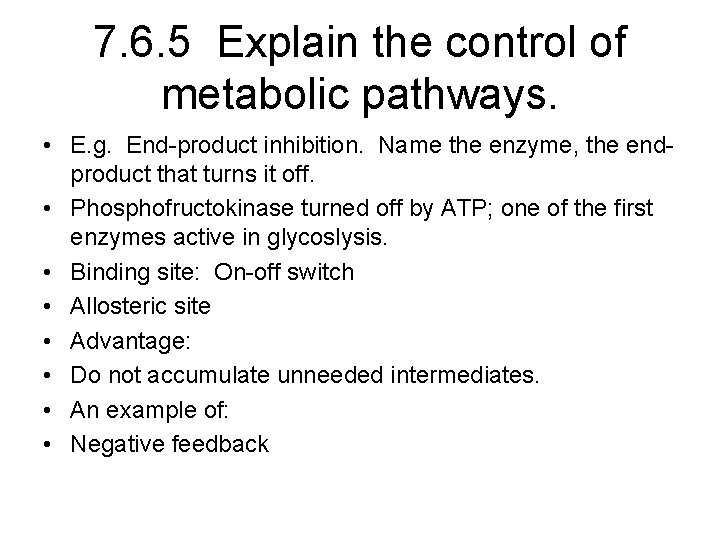
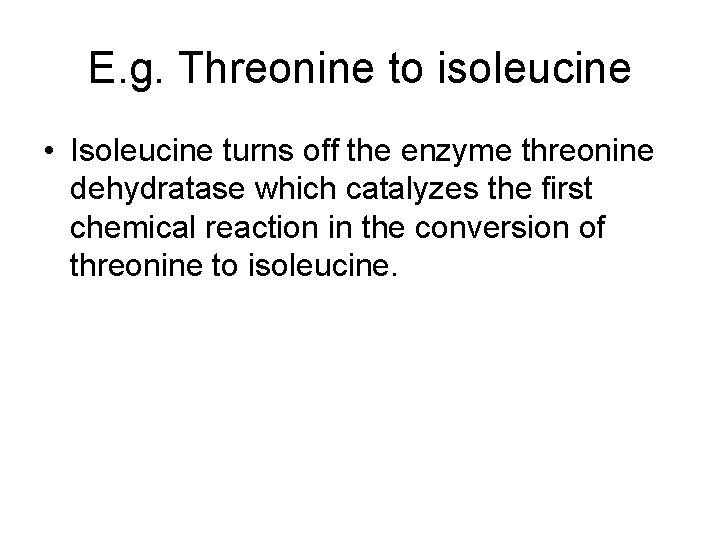
- Slides: 102
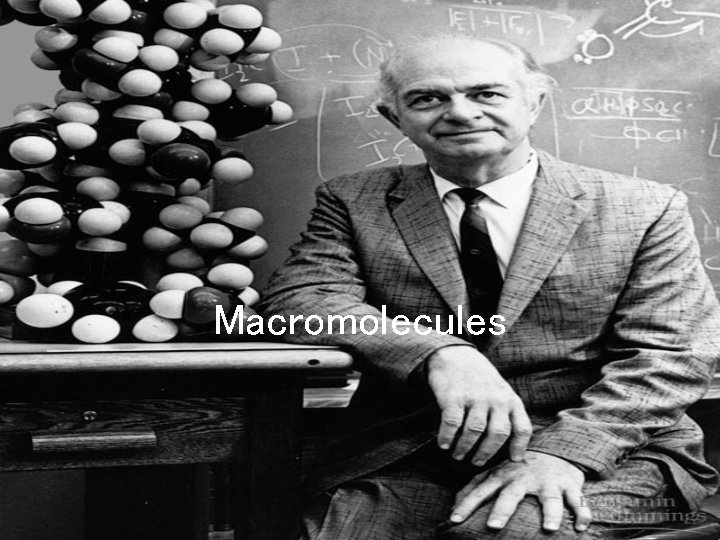
Macromolecules
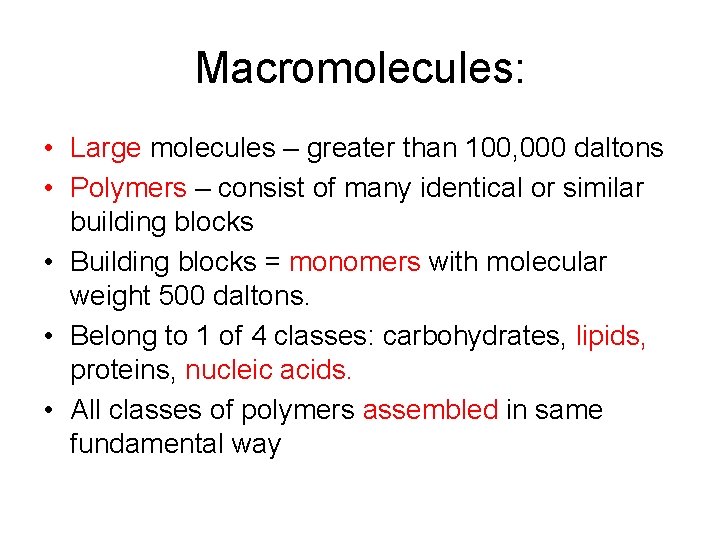
Macromolecules: • Large molecules – greater than 100, 000 daltons • Polymers – consist of many identical or similar building blocks • Building blocks = monomers with molecular weight 500 daltons. • Belong to 1 of 4 classes: carbohydrates, lipids, proteins, nucleic acids. • All classes of polymers assembled in same fundamental way
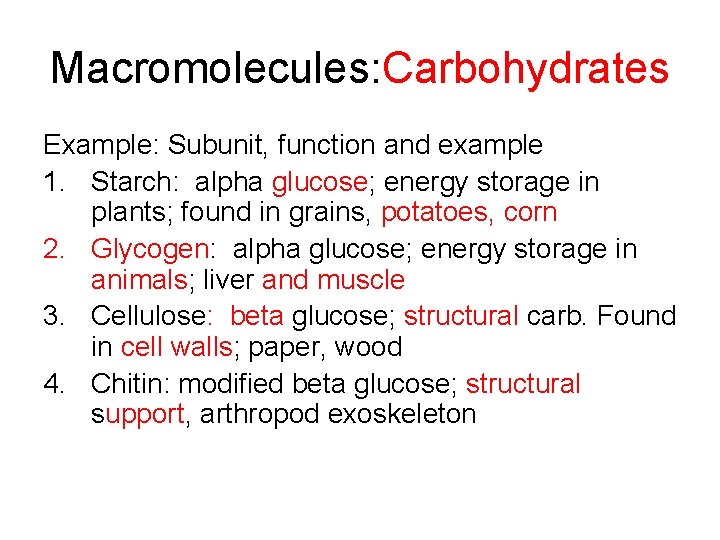
Macromolecules: Carbohydrates Example: Subunit, function and example 1. Starch: alpha glucose; energy storage in plants; found in grains, potatoes, corn 2. Glycogen: alpha glucose; energy storage in animals; liver and muscle 3. Cellulose: beta glucose; structural carb. Found in cell walls; paper, wood 4. Chitin: modified beta glucose; structural support, arthropod exoskeleton
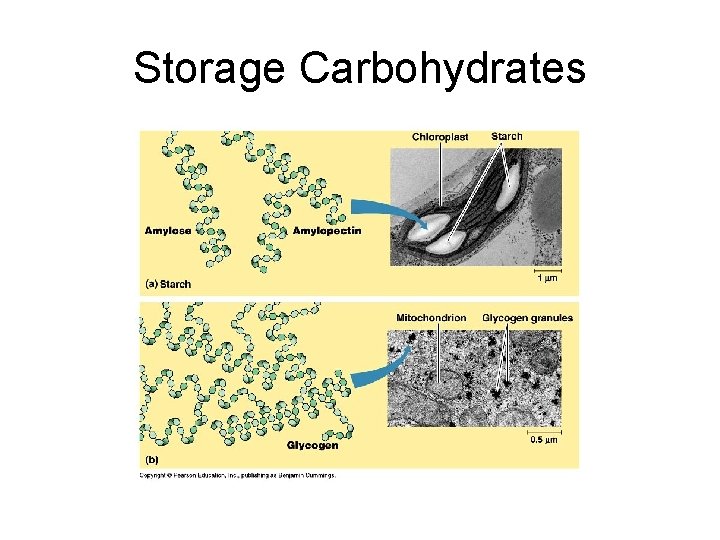
Storage Carbohydrates
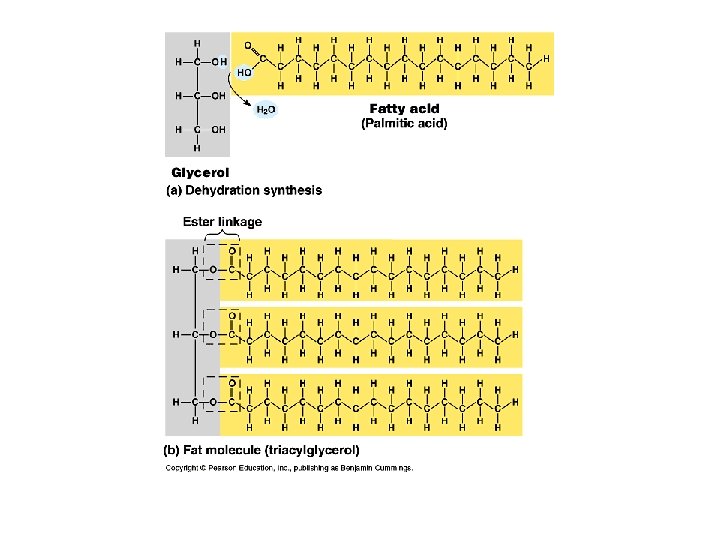
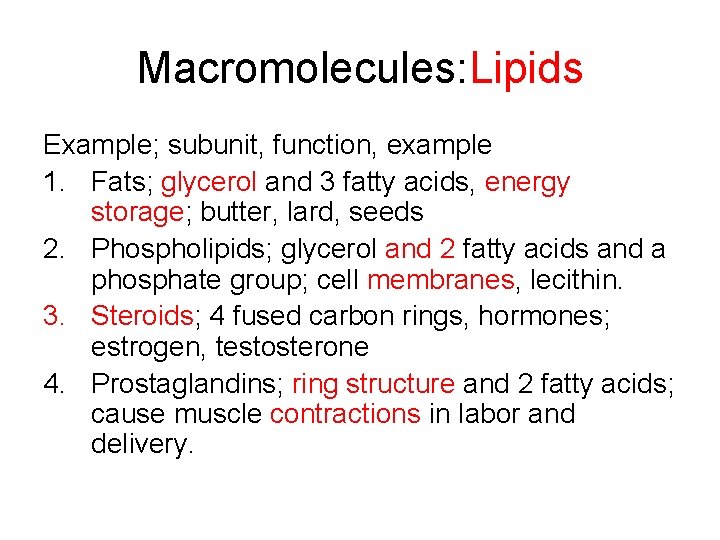
Macromolecules: Lipids Example; subunit, function, example 1. Fats; glycerol and 3 fatty acids, energy storage; butter, lard, seeds 2. Phospholipids; glycerol and 2 fatty acids and a phosphate group; cell membranes, lecithin. 3. Steroids; 4 fused carbon rings, hormones; estrogen, testosterone 4. Prostaglandins; ring structure and 2 fatty acids; cause muscle contractions in labor and delivery.
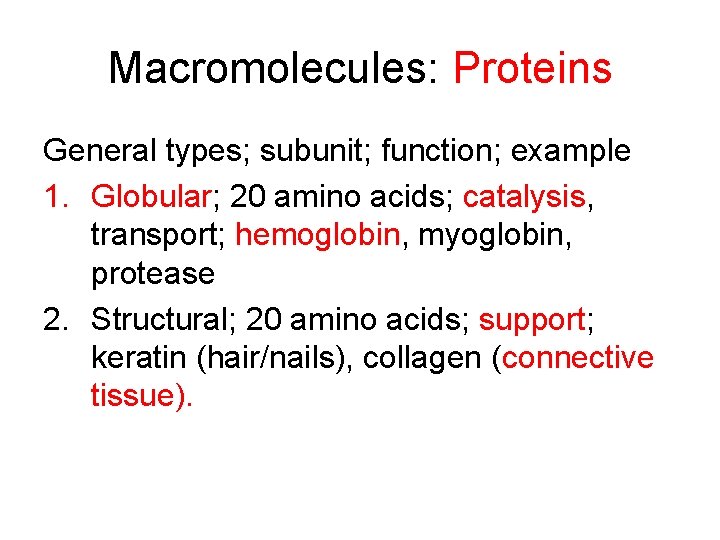
Macromolecules: Proteins General types; subunit; function; example 1. Globular; 20 amino acids; catalysis, transport; hemoglobin, myoglobin, protease 2. Structural; 20 amino acids; support; keratin (hair/nails), collagen (connective tissue).
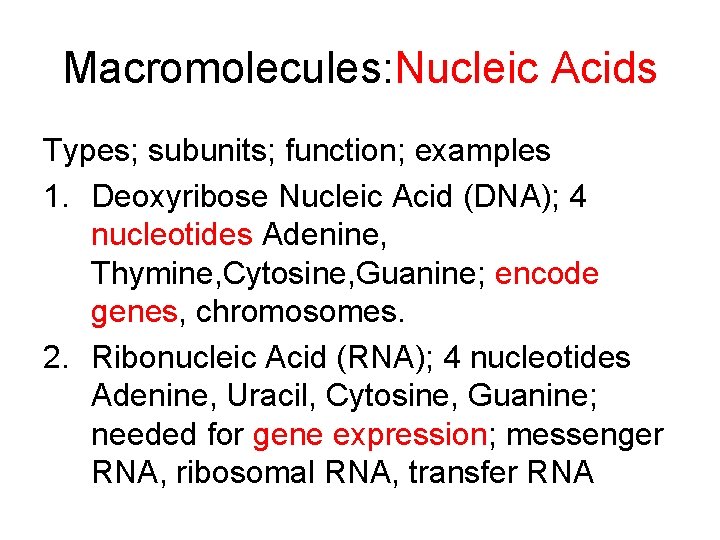
Macromolecules: Nucleic Acids Types; subunits; function; examples 1. Deoxyribose Nucleic Acid (DNA); 4 nucleotides Adenine, Thymine, Cytosine, Guanine; encode genes, chromosomes. 2. Ribonucleic Acid (RNA); 4 nucleotides Adenine, Uracil, Cytosine, Guanine; needed for gene expression; messenger RNA, ribosomal RNA, transfer RNA
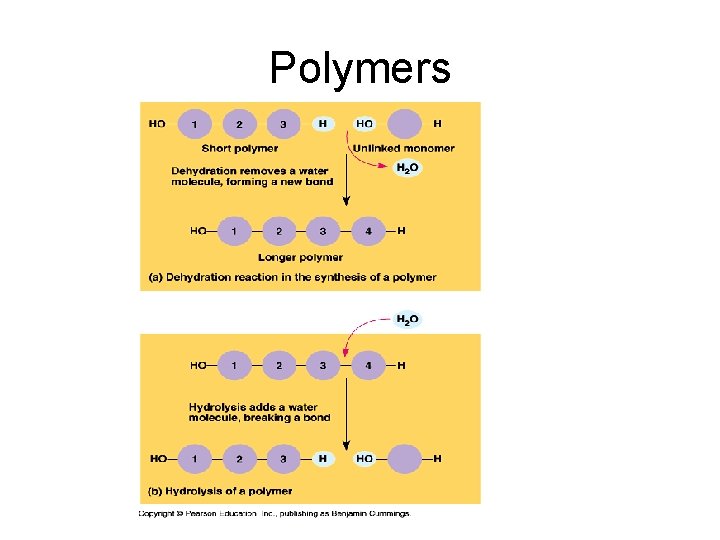
Polymers
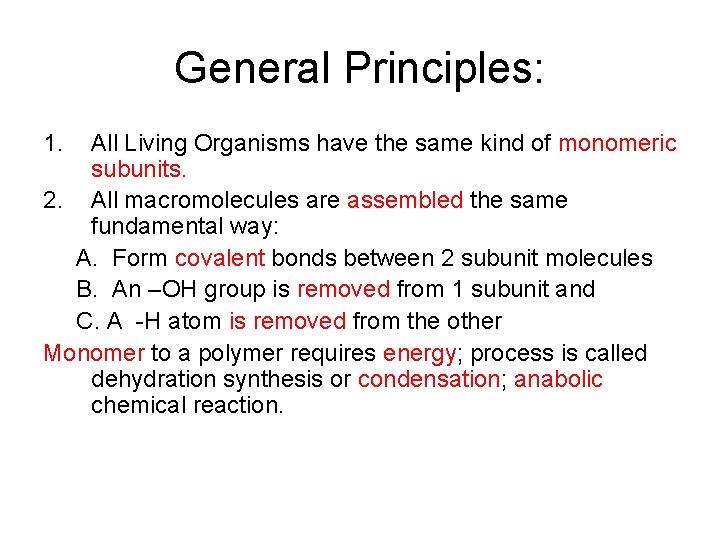
General Principles: 1. All Living Organisms have the same kind of monomeric subunits. 2. All macromolecules are assembled the same fundamental way: A. Form covalent bonds between 2 subunit molecules B. An –OH group is removed from 1 subunit and C. A -H atom is removed from the other Monomer to a polymer requires energy; process is called dehydration synthesis or condensation; anabolic chemical reaction.
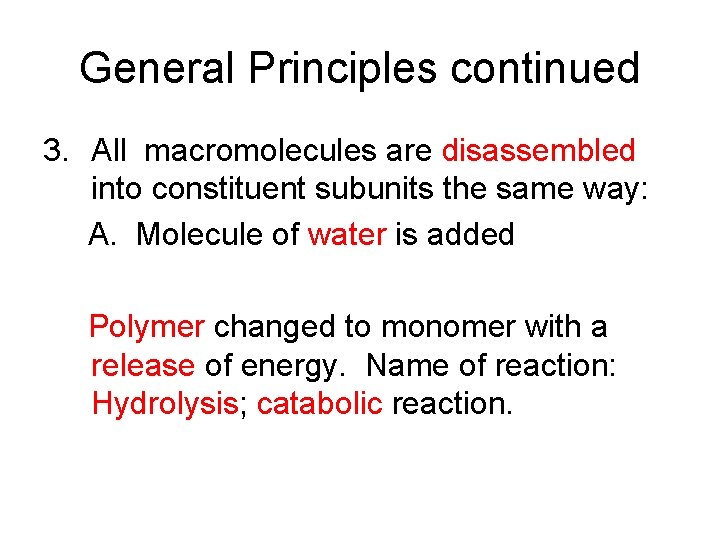
General Principles continued 3. All macromolecules are disassembled into constituent subunits the same way: A. Molecule of water is added Polymer changed to monomer with a release of energy. Name of reaction: Hydrolysis; catabolic reaction.
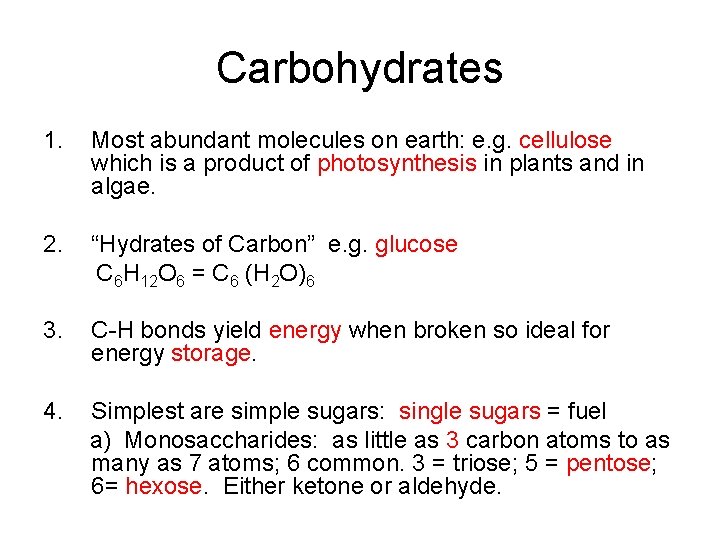
Carbohydrates 1. Most abundant molecules on earth: e. g. cellulose which is a product of photosynthesis in plants and in algae. 2. “Hydrates of Carbon” e. g. glucose C 6 H 12 O 6 = C 6 (H 2 O)6 3. C-H bonds yield energy when broken so ideal for energy storage. 4. Simplest are simple sugars: single sugars = fuel a) Monosaccharides: as little as 3 carbon atoms to as many as 7 atoms; 6 common. 3 = triose; 5 = pentose; 6= hexose. Either ketone or aldehyde.
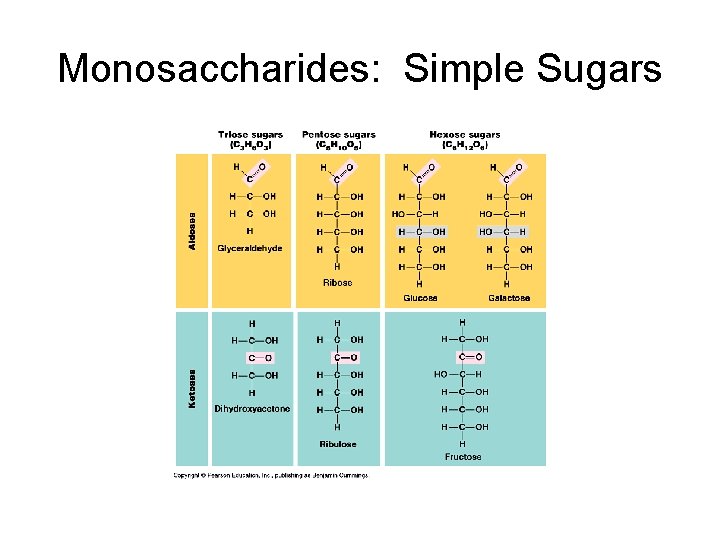
Monosaccharides: Simple Sugars
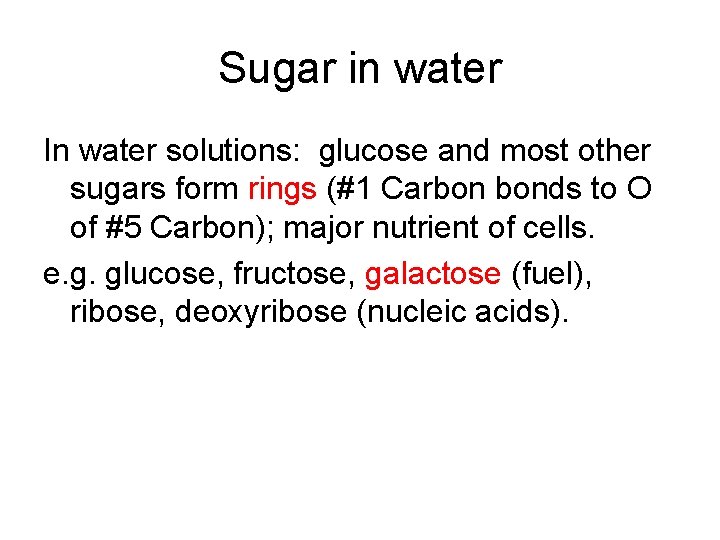
Sugar in water In water solutions: glucose and most other sugars form rings (#1 Carbon bonds to O of #5 Carbon); major nutrient of cells. e. g. glucose, fructose, galactose (fuel), ribose, deoxyribose (nucleic acids).
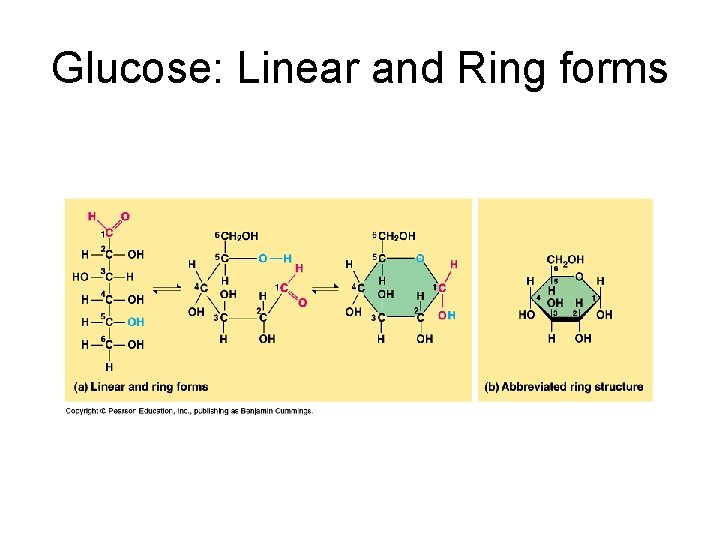
Glucose: Linear and Ring forms
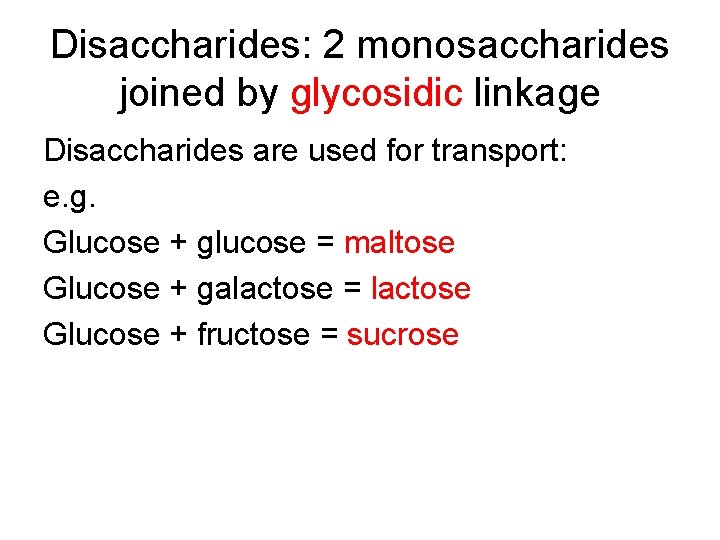
Disaccharides: 2 monosaccharides joined by glycosidic linkage Disaccharides are used for transport: e. g. Glucose + glucose = maltose Glucose + galactose = lactose Glucose + fructose = sucrose
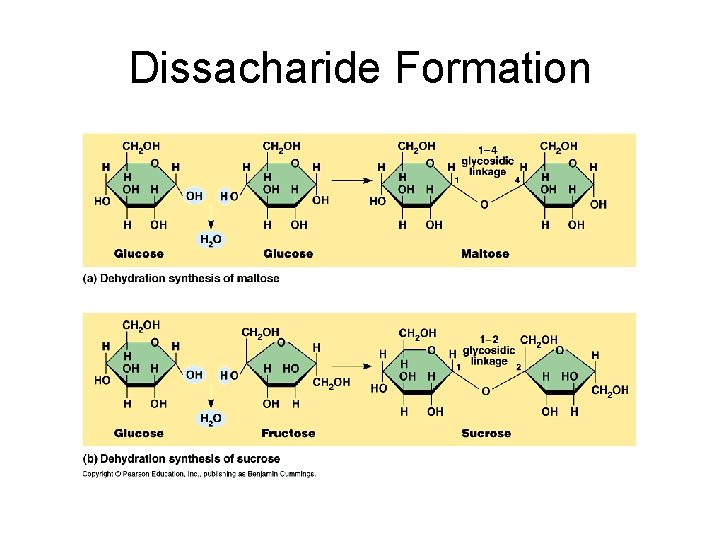
Dissacharide Formation
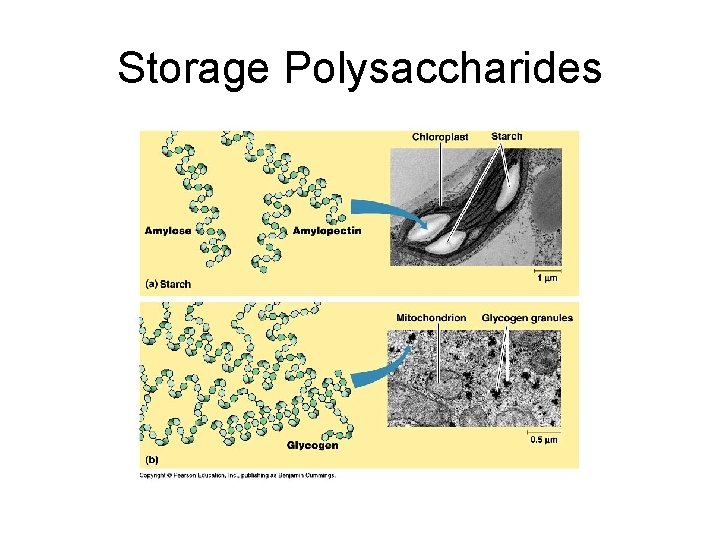
Storage Polysaccharides
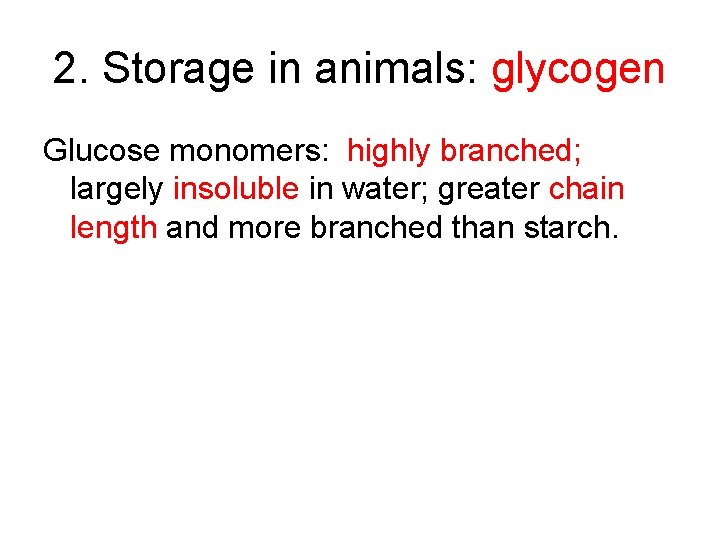
2. Storage in animals: glycogen Glucose monomers: highly branched; largely insoluble in water; greater chain length and more branched than starch.
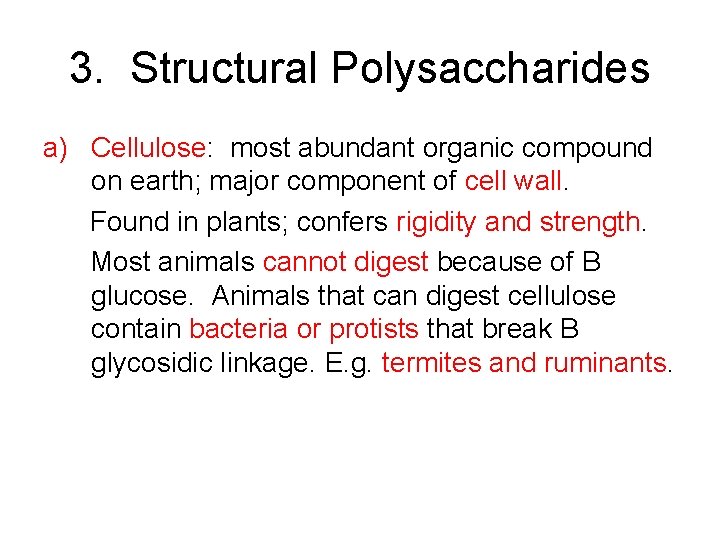
3. Structural Polysaccharides a) Cellulose: most abundant organic compound on earth; major component of cell wall. Found in plants; confers rigidity and strength. Most animals cannot digest because of B glucose. Animals that can digest cellulose contain bacteria or protists that break B glycosidic linkage. E. g. termites and ruminants.
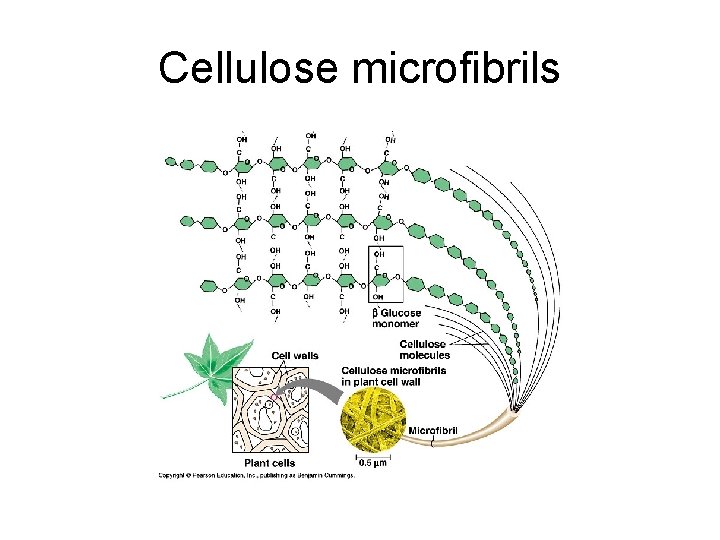
Cellulose microfibrils
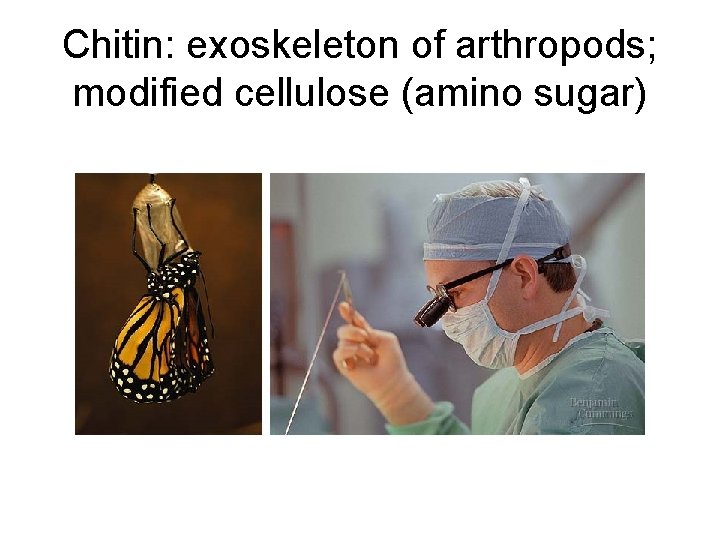
Chitin: exoskeleton of arthropods; modified cellulose (amino sugar)
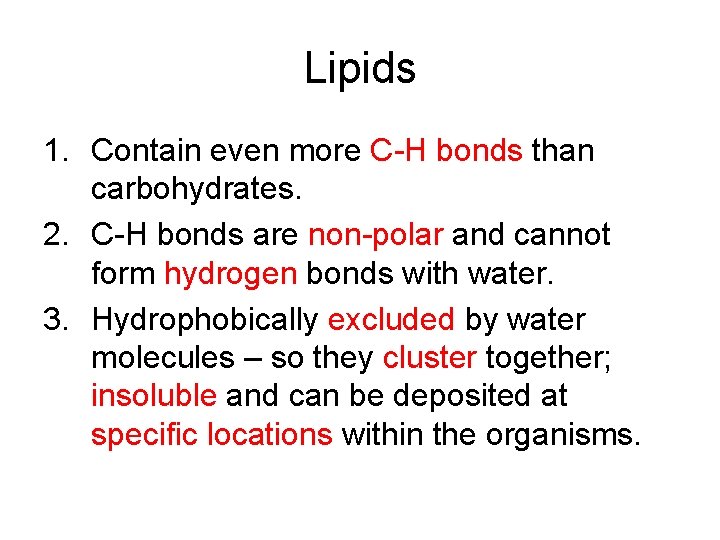
Lipids 1. Contain even more C-H bonds than carbohydrates. 2. C-H bonds are non-polar and cannot form hydrogen bonds with water. 3. Hydrophobically excluded by water molecules – so they cluster together; insoluble and can be deposited at specific locations within the organisms.
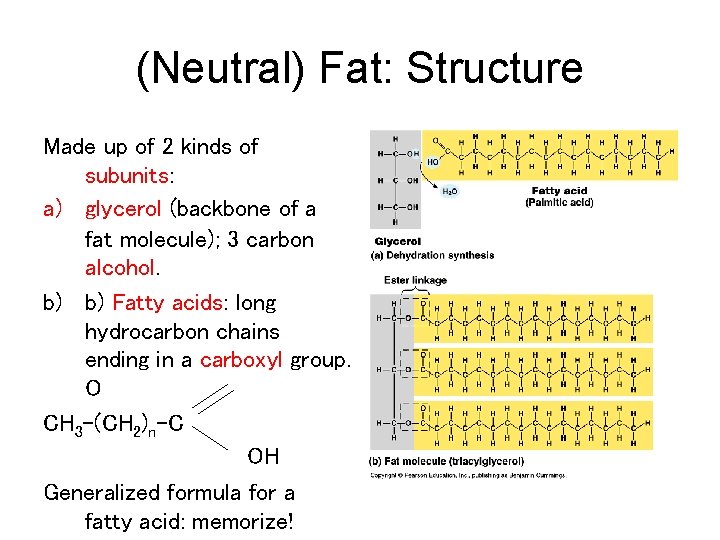
(Neutral) Fat: Structure Made up of 2 kinds of subunits: a) glycerol (backbone of a fat molecule); 3 carbon alcohol. b) b) Fatty acids: long hydrocarbon chains ending in a carboxyl group. O CH 3 -(CH 2)n-C OH Generalized formula for a fatty acid: memorize!
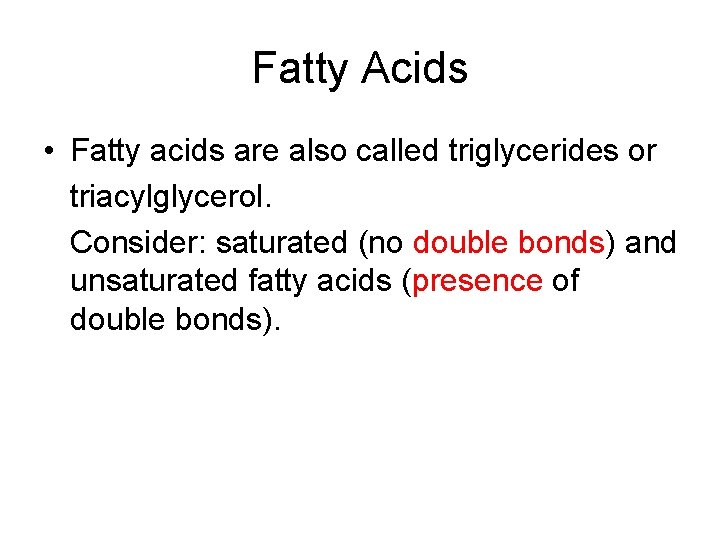
Fatty Acids • Fatty acids are also called triglycerides or triacylglycerol. Consider: saturated (no double bonds) and unsaturated fatty acids (presence of double bonds).
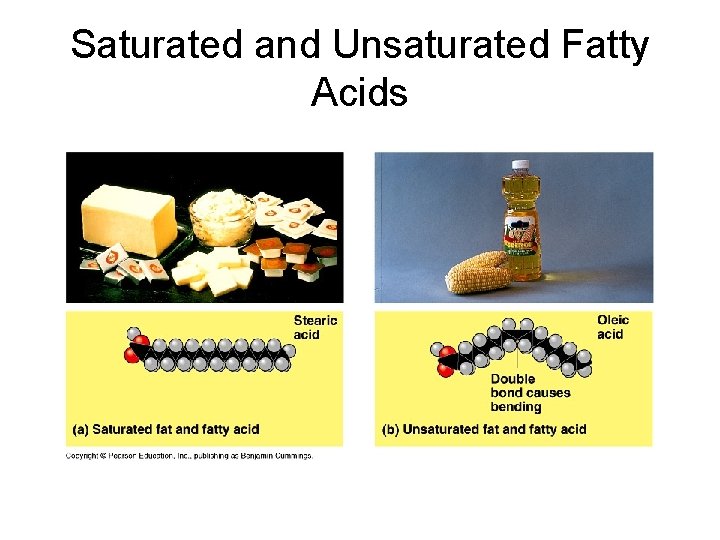
Saturated and Unsaturated Fatty Acids
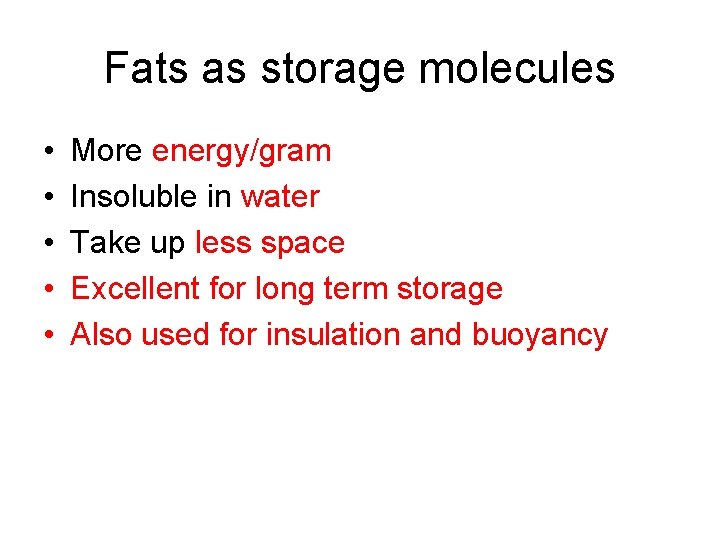
Fats as storage molecules • • • More energy/gram Insoluble in water Take up less space Excellent for long term storage Also used for insulation and buoyancy
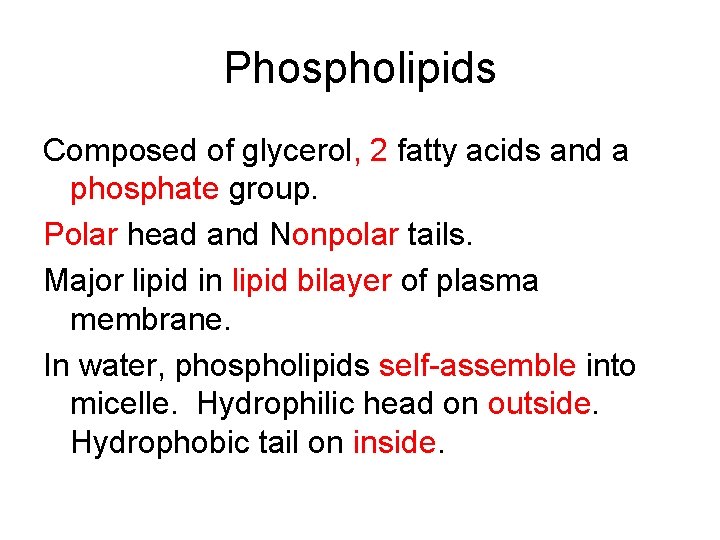
Phospholipids Composed of glycerol, 2 fatty acids and a phosphate group. Polar head and Nonpolar tails. Major lipid in lipid bilayer of plasma membrane. In water, phospholipids self-assemble into micelle. Hydrophilic head on outside. Hydrophobic tail on inside.
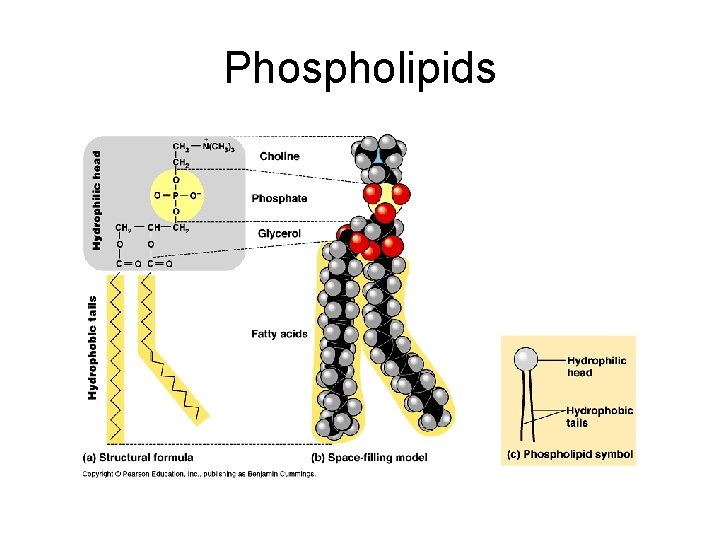
Phospholipids
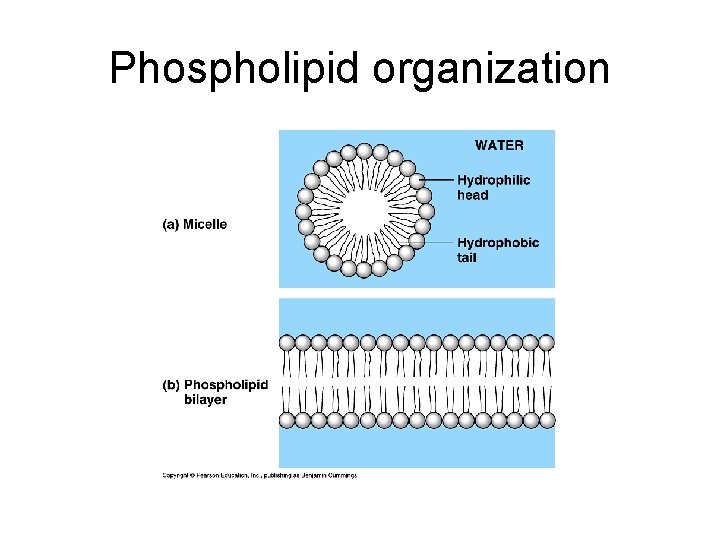
Phospholipid organization
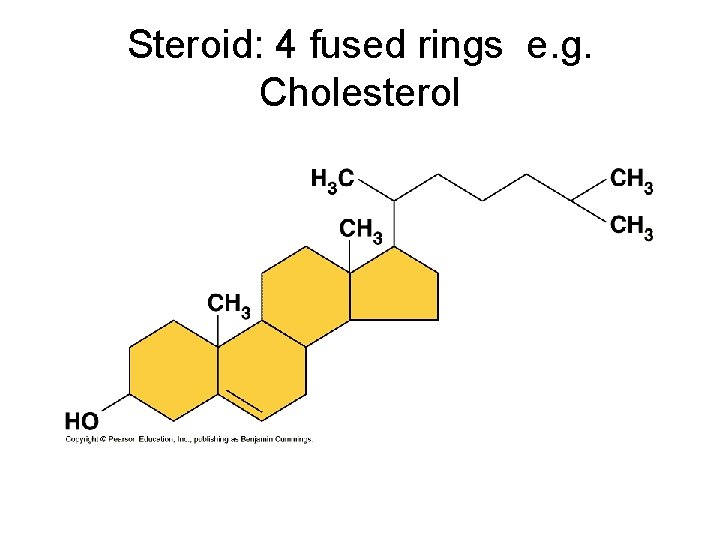
Steroid: 4 fused rings e. g. Cholesterol
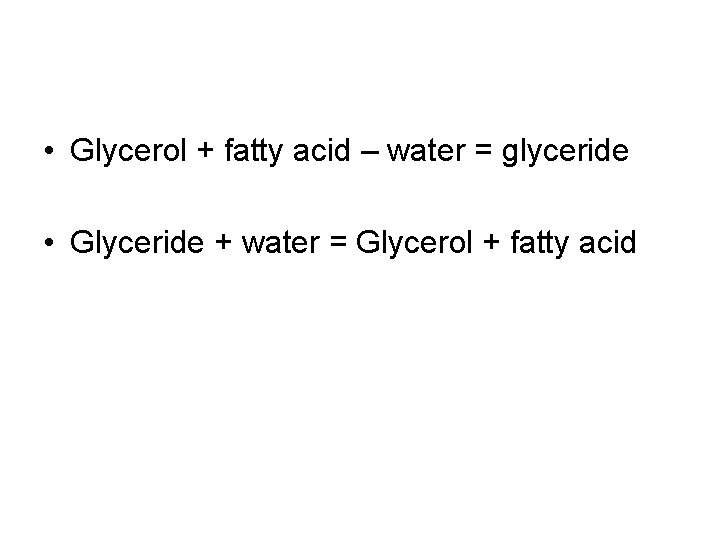
• Glycerol + fatty acid – water = glyceride • Glyceride + water = Glycerol + fatty acid
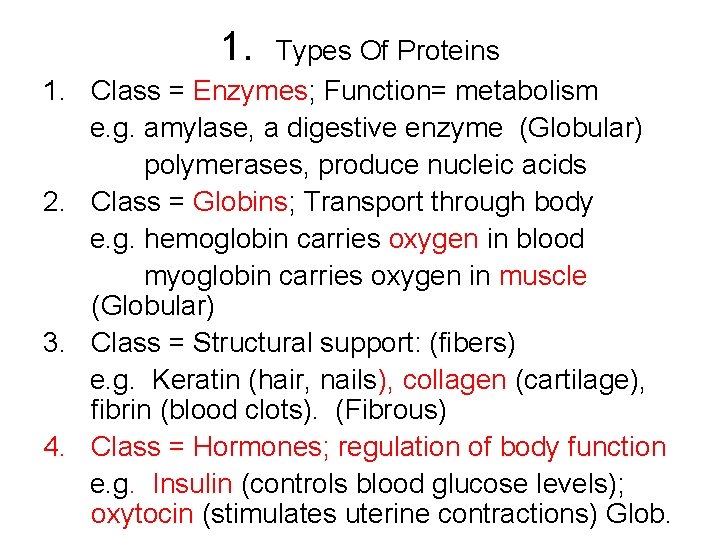
1. 2. 3. 4. Types Of Proteins Class = Enzymes; Function= metabolism e. g. amylase, a digestive enzyme (Globular) polymerases, produce nucleic acids Class = Globins; Transport through body e. g. hemoglobin carries oxygen in blood myoglobin carries oxygen in muscle (Globular) Class = Structural support: (fibers) e. g. Keratin (hair, nails), collagen (cartilage), fibrin (blood clots). (Fibrous) Class = Hormones; regulation of body function e. g. Insulin (controls blood glucose levels); oxytocin (stimulates uterine contractions) Glob.
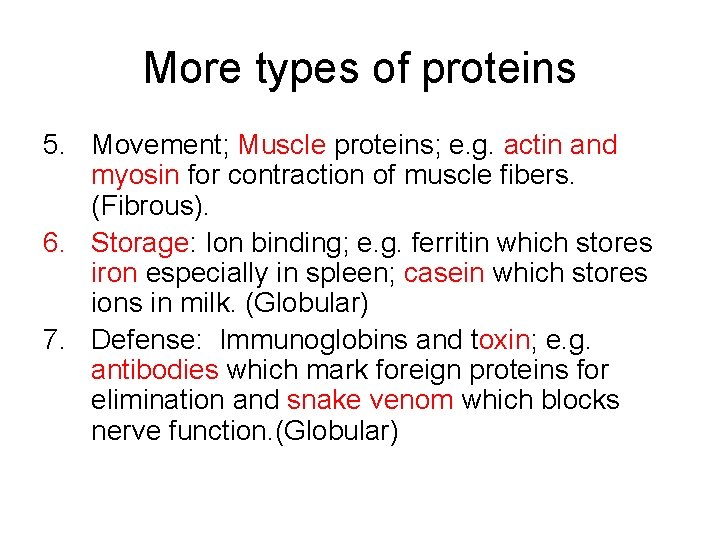
More types of proteins 5. Movement; Muscle proteins; e. g. actin and myosin for contraction of muscle fibers. (Fibrous). 6. Storage: Ion binding; e. g. ferritin which stores iron especially in spleen; casein which stores ions in milk. (Globular) 7. Defense: Immunoglobins and toxin; e. g. antibodies which mark foreign proteins for elimination and snake venom which blocks nerve function. (Globular)
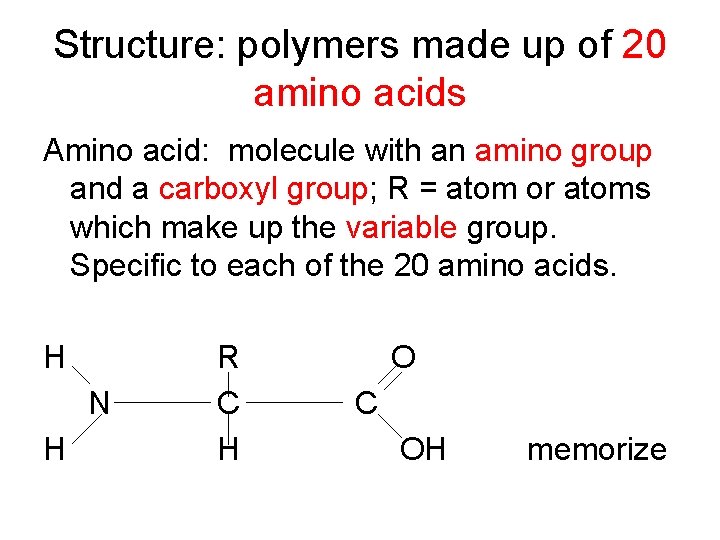
Structure: polymers made up of 20 amino acids Amino acid: molecule with an amino group and a carboxyl group; R = atom or atoms which make up the variable group. Specific to each of the 20 amino acids. H N H R C H O C OH memorize
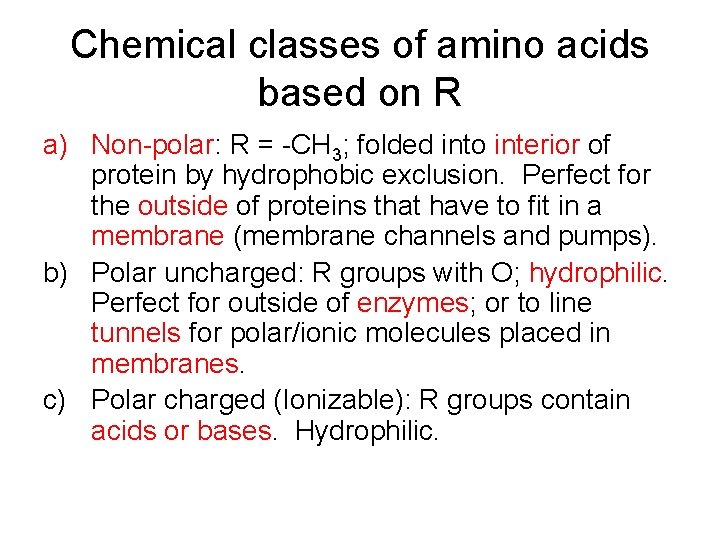
Chemical classes of amino acids based on R a) Non-polar: R = -CH 3; folded into interior of protein by hydrophobic exclusion. Perfect for the outside of proteins that have to fit in a membrane (membrane channels and pumps). b) Polar uncharged: R groups with O; hydrophilic. Perfect for outside of enzymes; or to line tunnels for polar/ionic molecules placed in membranes. c) Polar charged (Ionizable): R groups contain acids or bases. Hydrophilic.
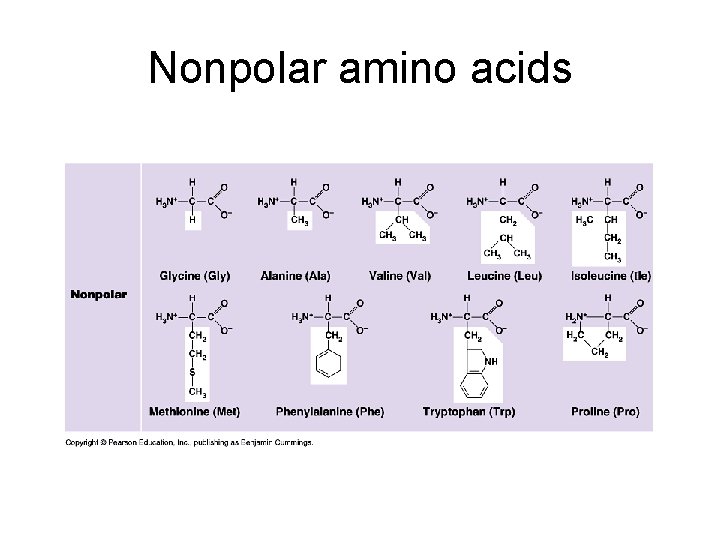
Nonpolar amino acids
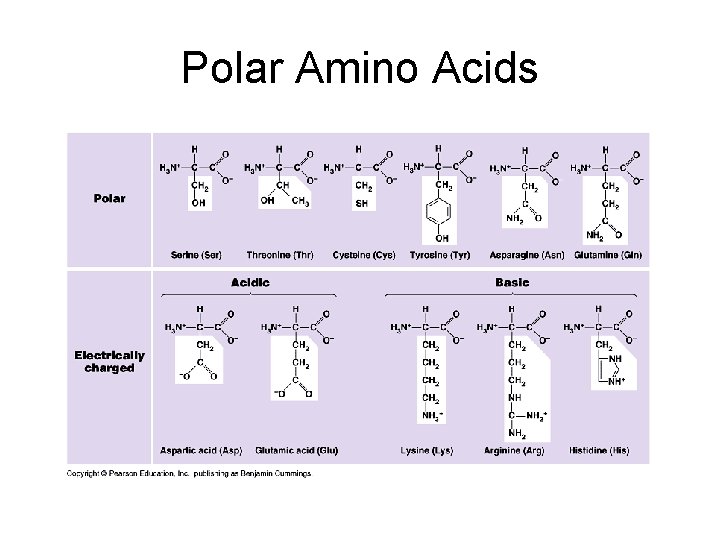
Polar Amino Acids
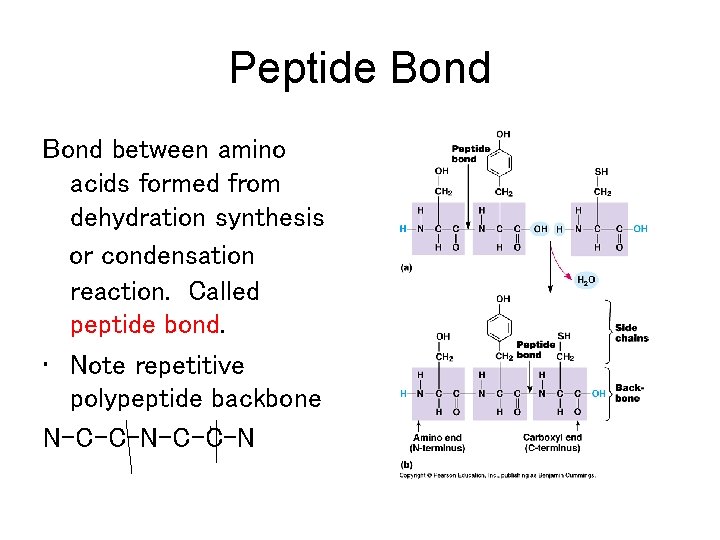
Peptide Bond between amino acids formed from dehydration synthesis or condensation reaction. Called peptide bond. • Note repetitive polypeptide backbone N-C-C-N
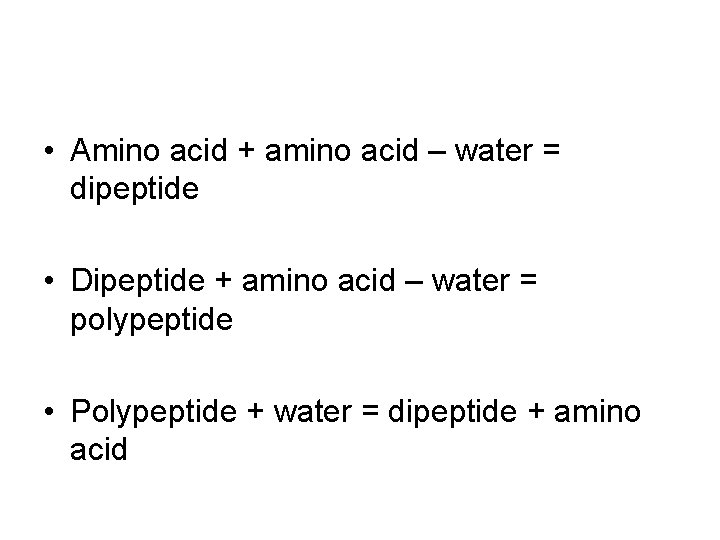
• Amino acid + amino acid – water = dipeptide • Dipeptide + amino acid – water = polypeptide • Polypeptide + water = dipeptide + amino acid
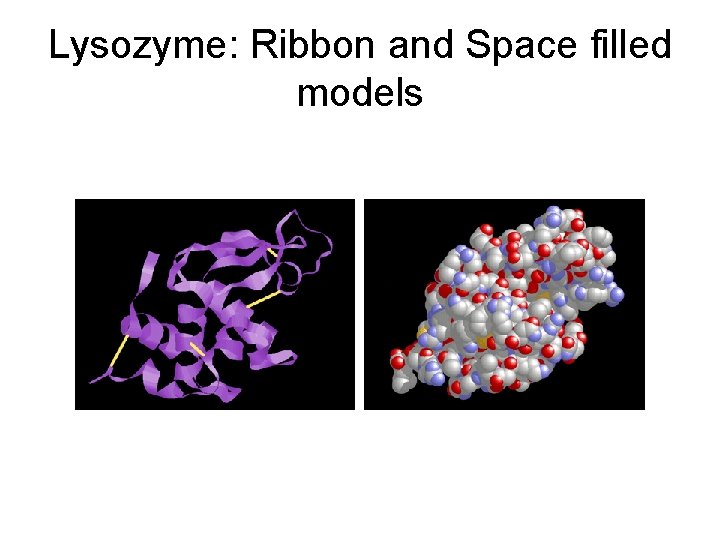
Lysozyme: Ribbon and Space filled models
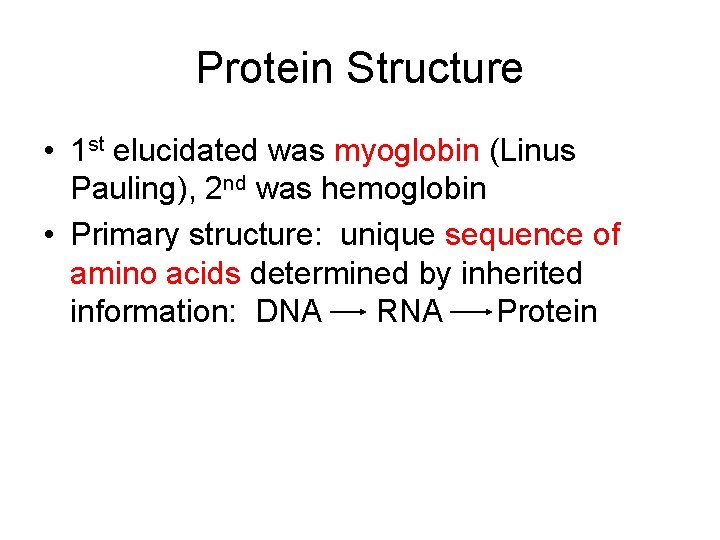
Protein Structure • 1 st elucidated was myoglobin (Linus Pauling), 2 nd was hemoglobin • Primary structure: unique sequence of amino acids determined by inherited information: DNA RNA Protein
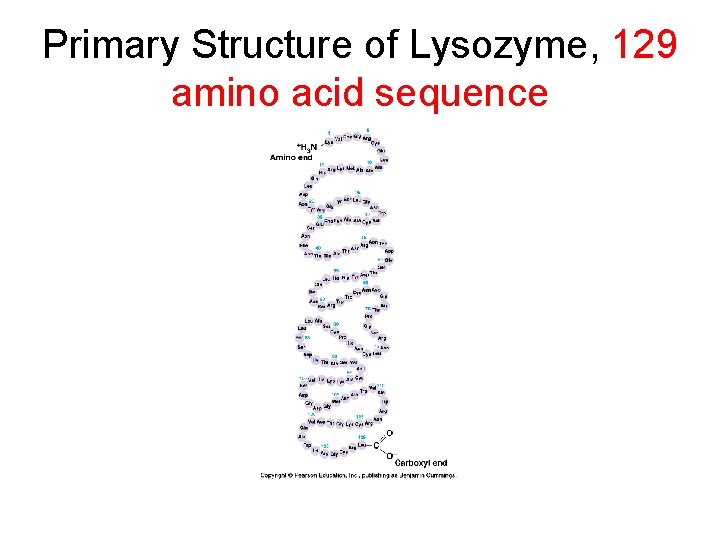
Primary Structure of Lysozyme, 129 amino acid sequence
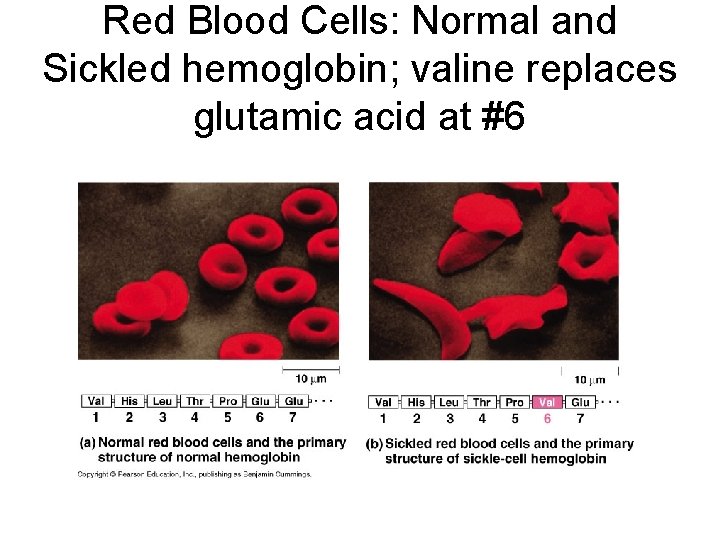
Red Blood Cells: Normal and Sickled hemoglobin; valine replaces glutamic acid at #6
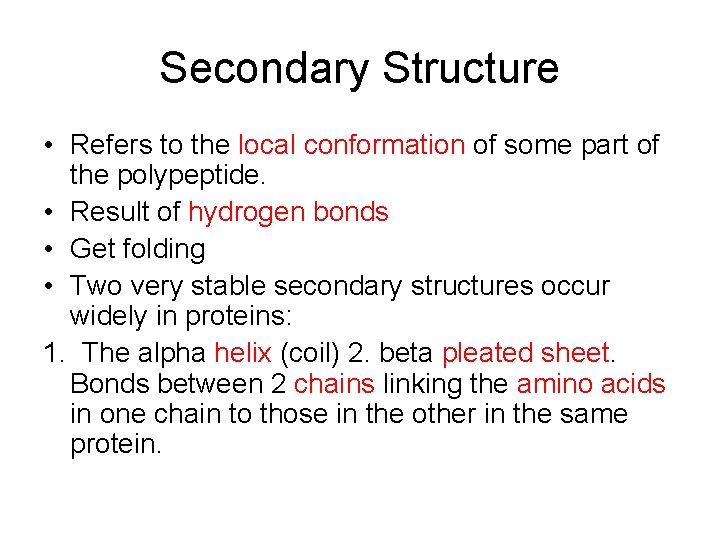
Secondary Structure • Refers to the local conformation of some part of the polypeptide. • Result of hydrogen bonds • Get folding • Two very stable secondary structures occur widely in proteins: 1. The alpha helix (coil) 2. beta pleated sheet. Bonds between 2 chains linking the amino acids in one chain to those in the other in the same protein.
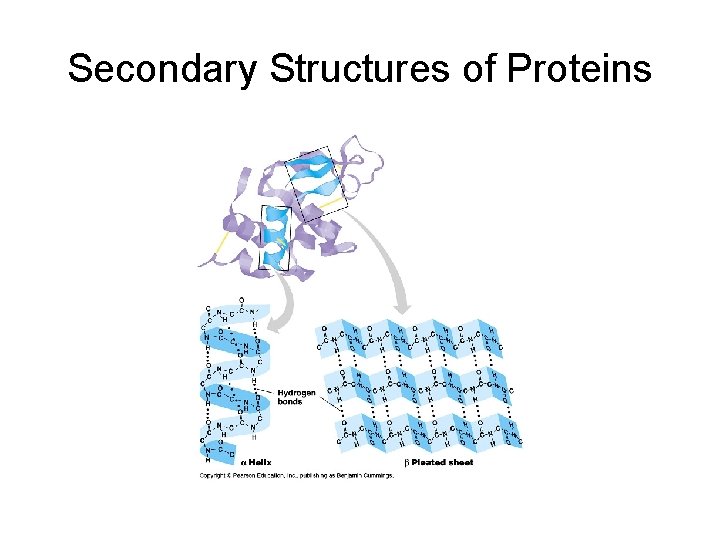
Secondary Structures of Proteins
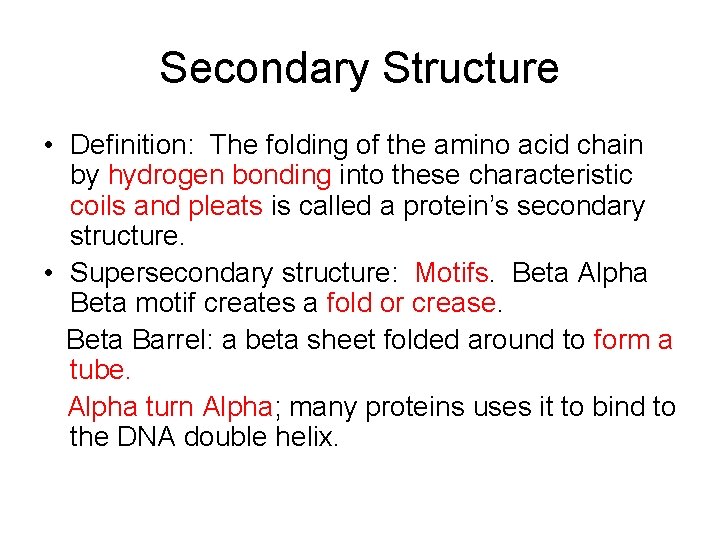
Secondary Structure • Definition: The folding of the amino acid chain by hydrogen bonding into these characteristic coils and pleats is called a protein’s secondary structure. • Supersecondary structure: Motifs. Beta Alpha Beta motif creates a fold or crease. Beta Barrel: a beta sheet folded around to form a tube. Alpha turn Alpha; many proteins uses it to bind to the DNA double helix.
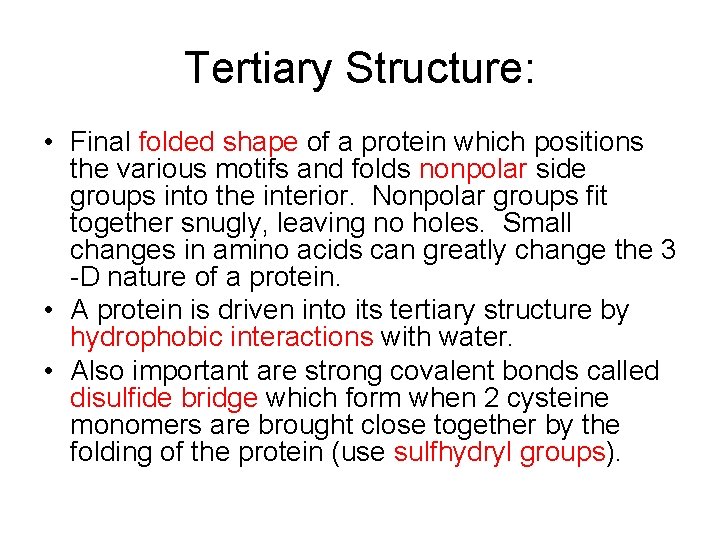
Tertiary Structure: • Final folded shape of a protein which positions the various motifs and folds nonpolar side groups into the interior. Nonpolar groups fit together snugly, leaving no holes. Small changes in amino acids can greatly change the 3 -D nature of a protein. • A protein is driven into its tertiary structure by hydrophobic interactions with water. • Also important are strong covalent bonds called disulfide bridge which form when 2 cysteine monomers are brought close together by the folding of the protein (use sulfhydryl groups).
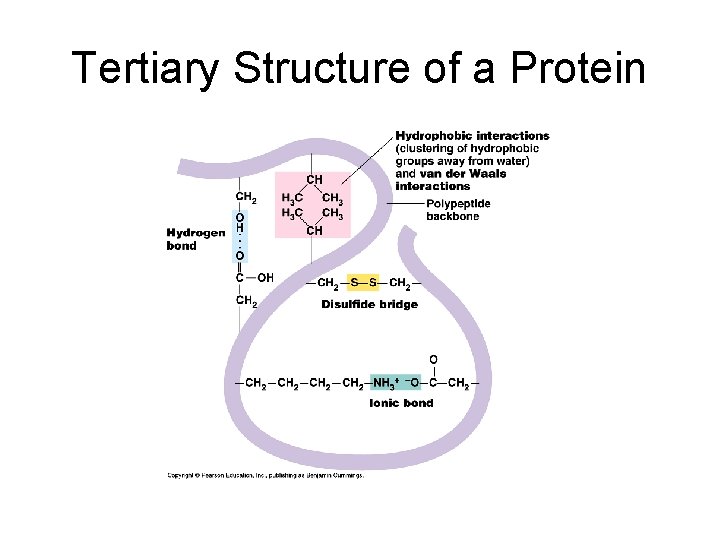
Tertiary Structure of a Protein
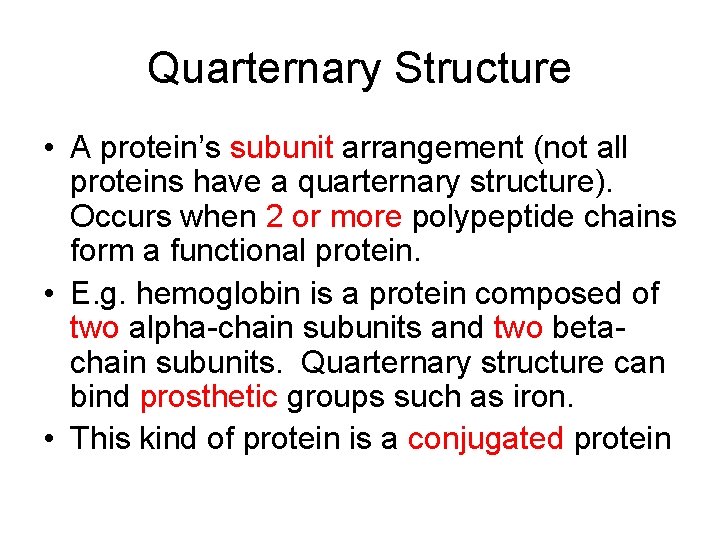
Quarternary Structure • A protein’s subunit arrangement (not all proteins have a quarternary structure). Occurs when 2 or more polypeptide chains form a functional protein. • E. g. hemoglobin is a protein composed of two alpha-chain subunits and two betachain subunits. Quarternary structure can bind prosthetic groups such as iron. • This kind of protein is a conjugated protein
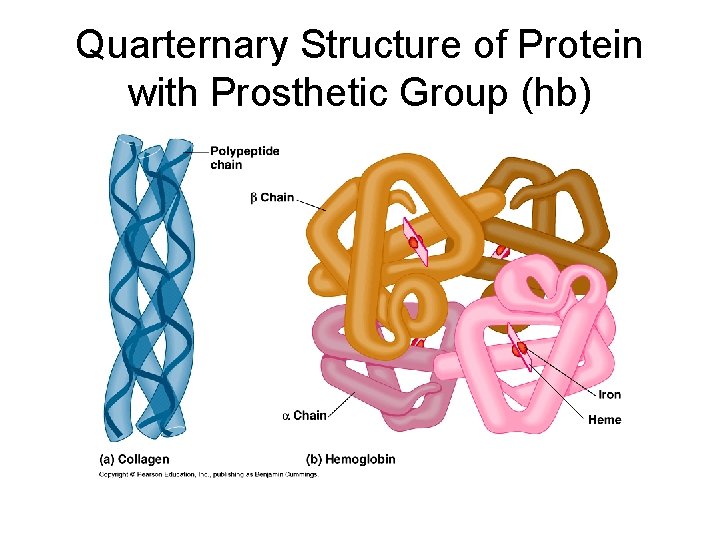
Quarternary Structure of Protein with Prosthetic Group (hb)
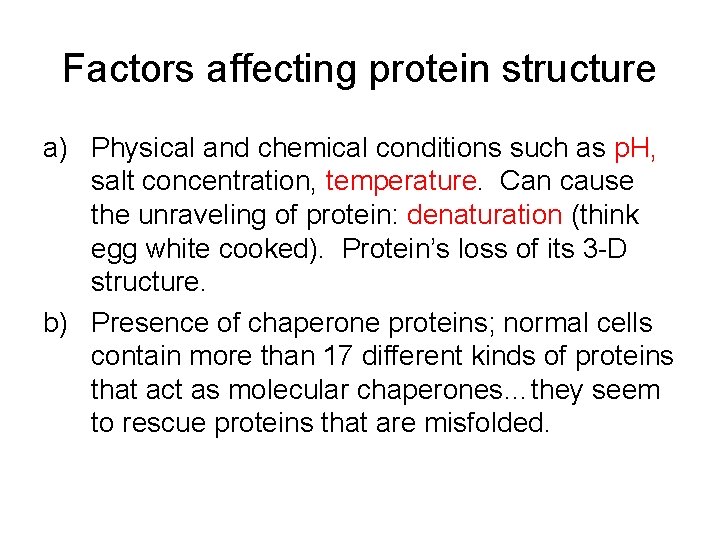
Factors affecting protein structure a) Physical and chemical conditions such as p. H, salt concentration, temperature. Can cause the unraveling of protein: denaturation (think egg white cooked). Protein’s loss of its 3 -D structure. b) Presence of chaperone proteins; normal cells contain more than 17 different kinds of proteins that act as molecular chaperones…they seem to rescue proteins that are misfolded.
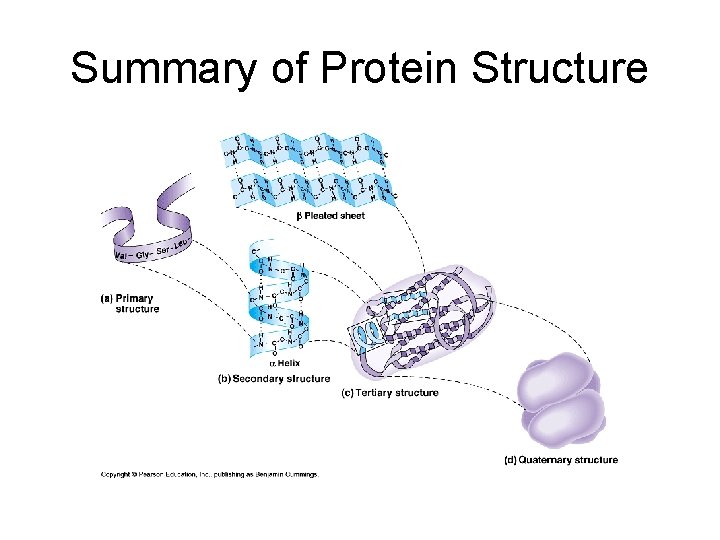
Summary of Protein Structure
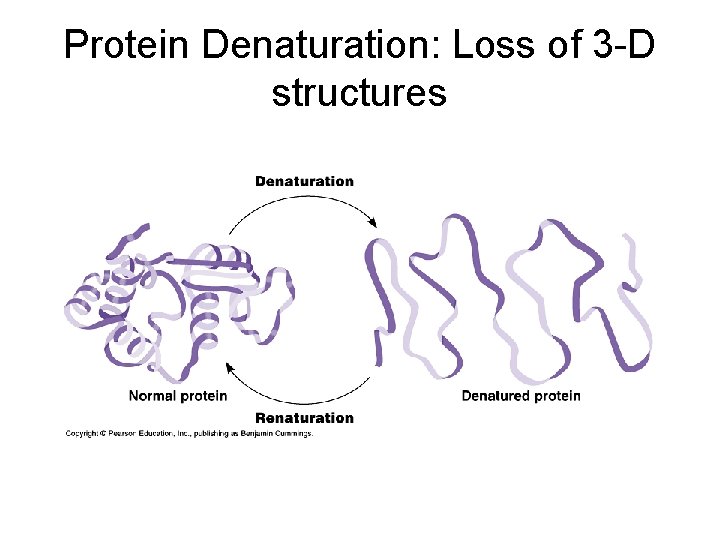
Protein Denaturation: Loss of 3 -D structures
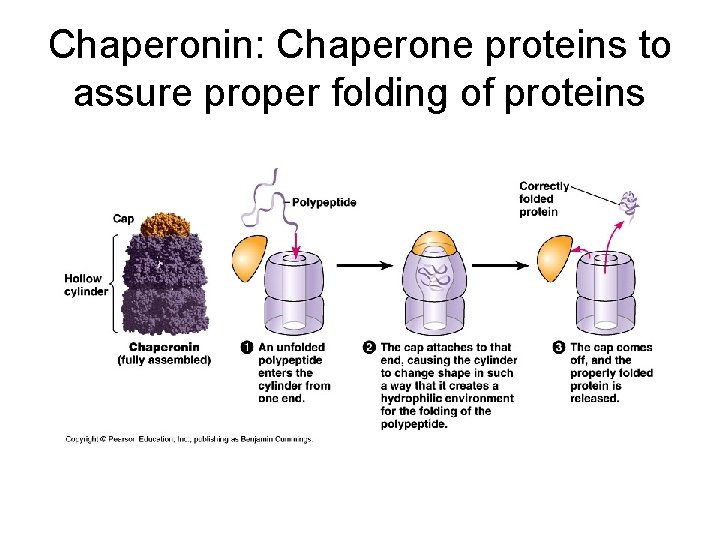
Chaperonin: Chaperone proteins to assure proper folding of proteins
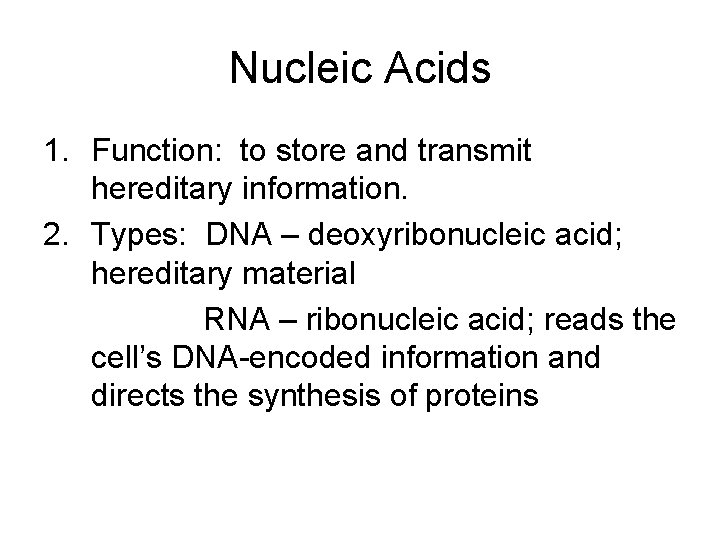
Nucleic Acids 1. Function: to store and transmit hereditary information. 2. Types: DNA – deoxyribonucleic acid; hereditary material RNA – ribonucleic acid; reads the cell’s DNA-encoded information and directs the synthesis of proteins
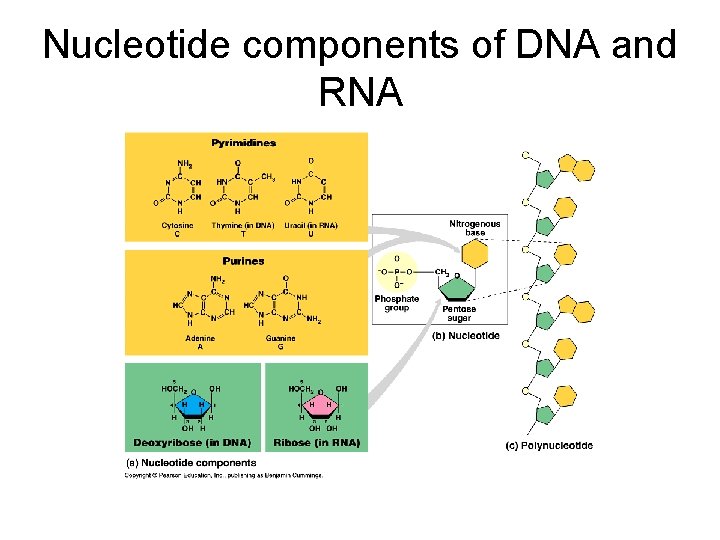
Nucleotide components of DNA and RNA
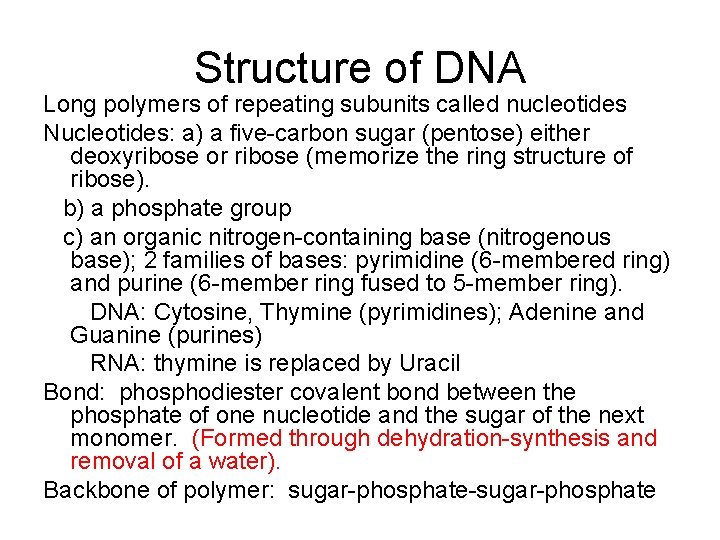
Structure of DNA Long polymers of repeating subunits called nucleotides Nucleotides: a) a five-carbon sugar (pentose) either deoxyribose or ribose (memorize the ring structure of ribose). b) a phosphate group c) an organic nitrogen-containing base (nitrogenous base); 2 families of bases: pyrimidine (6 -membered ring) and purine (6 -member ring fused to 5 -member ring). DNA: Cytosine, Thymine (pyrimidines); Adenine and Guanine (purines) RNA: thymine is replaced by Uracil Bond: phosphodiester covalent bond between the phosphate of one nucleotide and the sugar of the next monomer. (Formed through dehydration-synthesis and removal of a water). Backbone of polymer: sugar-phosphate-sugar-phosphate
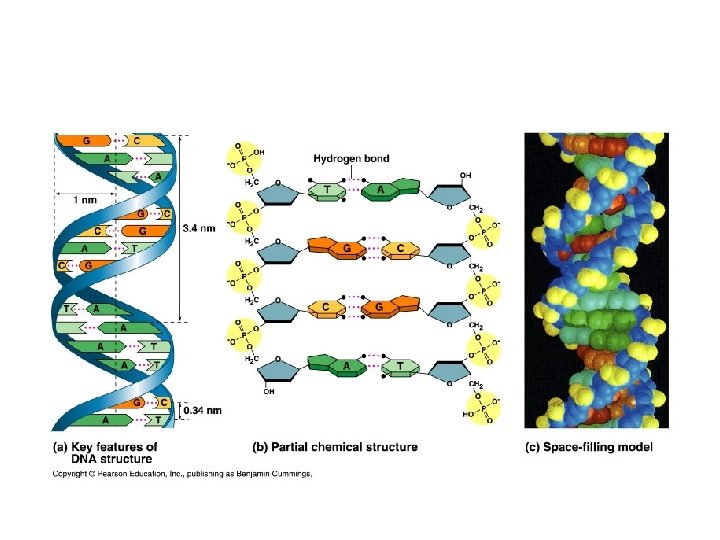
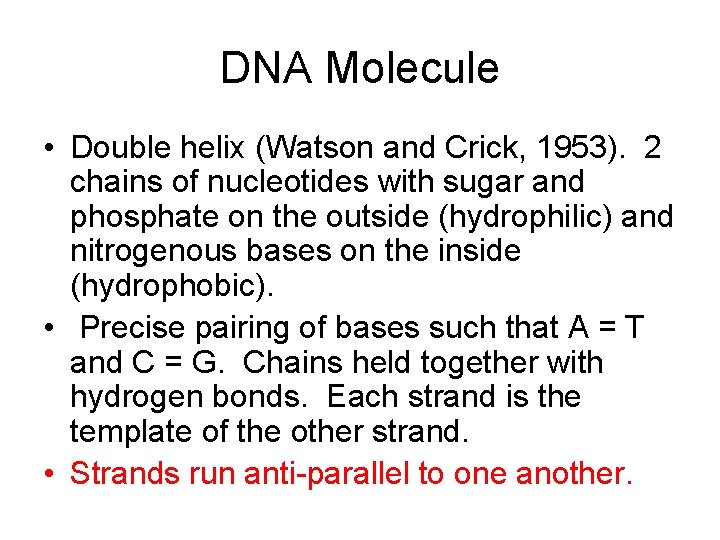
DNA Molecule • Double helix (Watson and Crick, 1953). 2 chains of nucleotides with sugar and phosphate on the outside (hydrophilic) and nitrogenous bases on the inside (hydrophobic). • Precise pairing of bases such that A = T and C = G. Chains held together with hydrogen bonds. Each strand is the template of the other strand. • Strands run anti-parallel to one another.
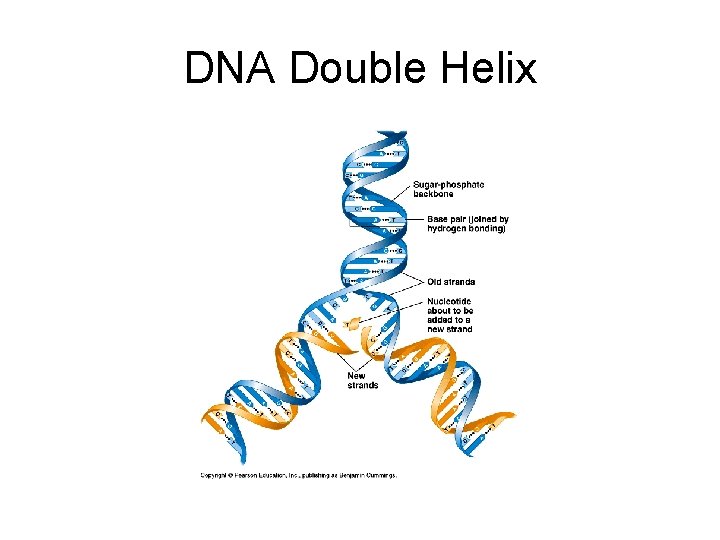
DNA Double Helix
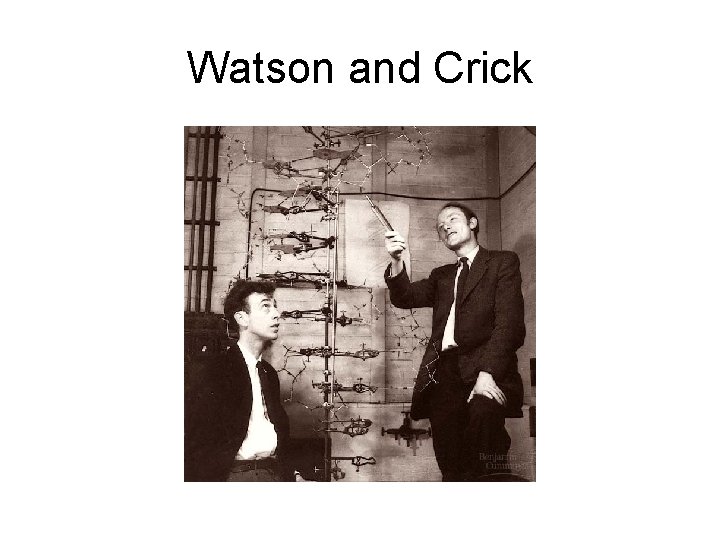
Watson and Crick
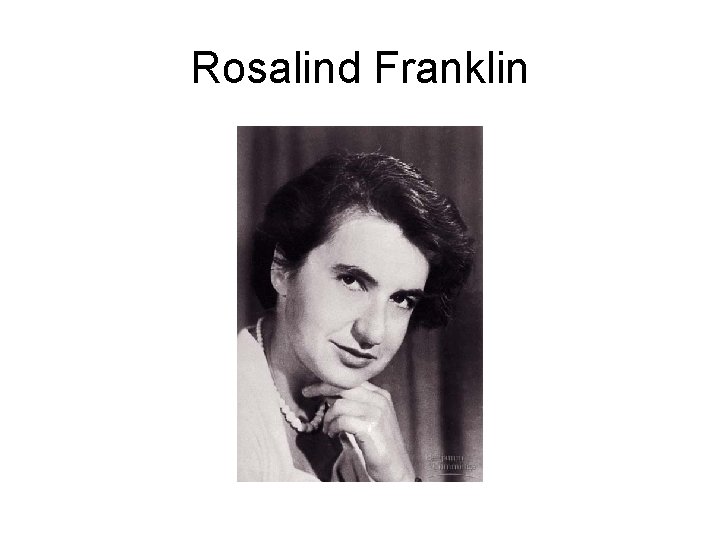
Rosalind Franklin
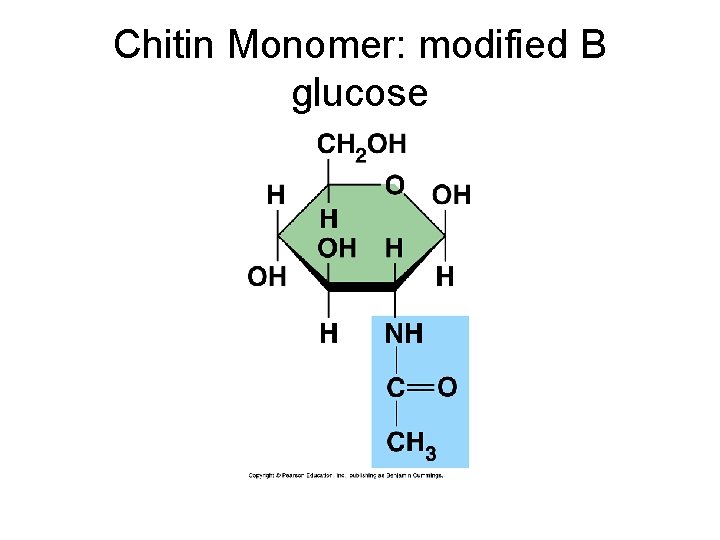
Chitin Monomer: modified B glucose
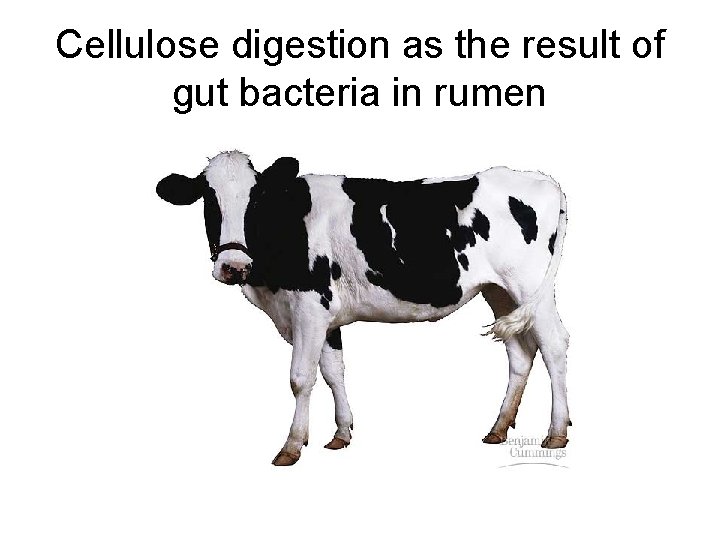
Cellulose digestion as the result of gut bacteria in rumen
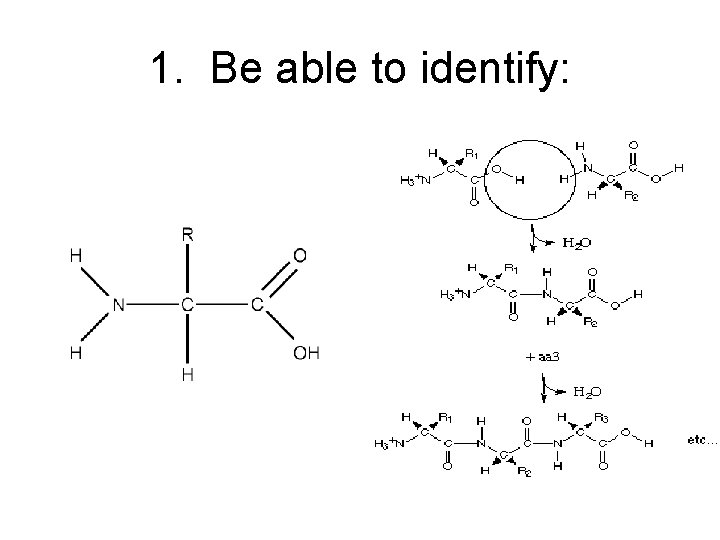
1. Be able to identify:
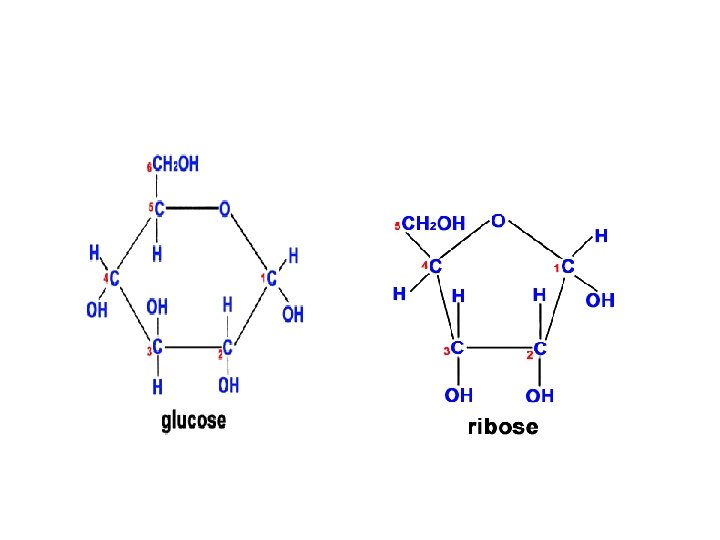
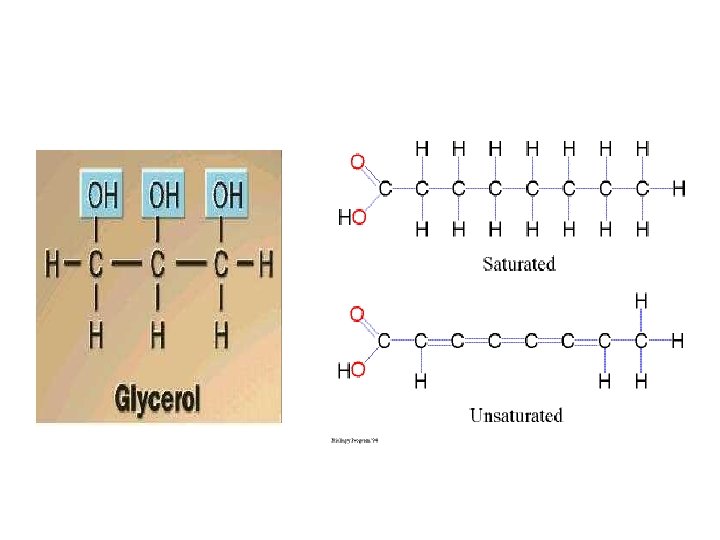
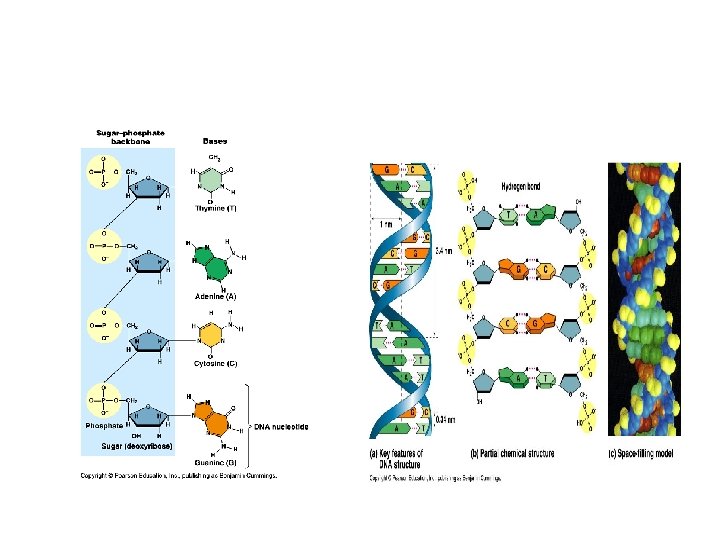
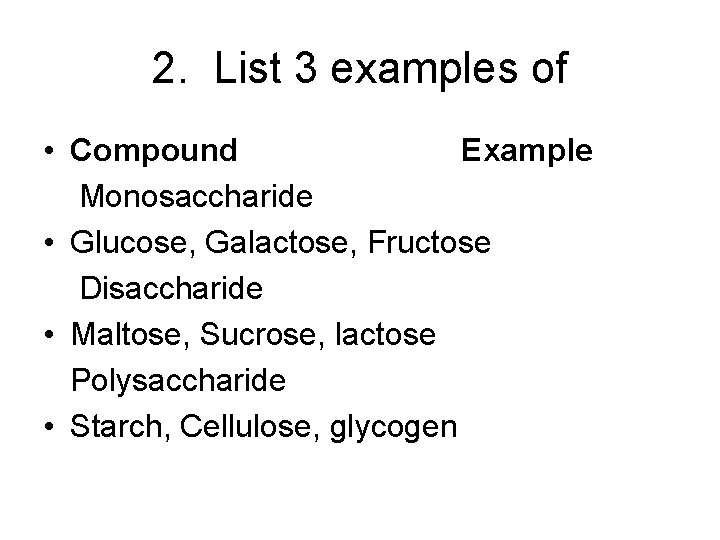
2. List 3 examples of • Compound Example Monosaccharide • Glucose, Galactose, Fructose Disaccharide • Maltose, Sucrose, lactose Polysaccharide • Starch, Cellulose, glycogen
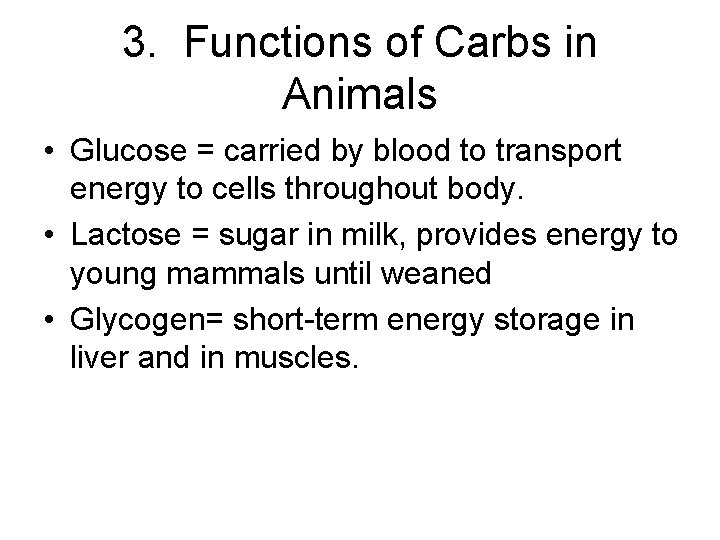
3. Functions of Carbs in Animals • Glucose = carried by blood to transport energy to cells throughout body. • Lactose = sugar in milk, provides energy to young mammals until weaned • Glycogen= short-term energy storage in liver and in muscles.
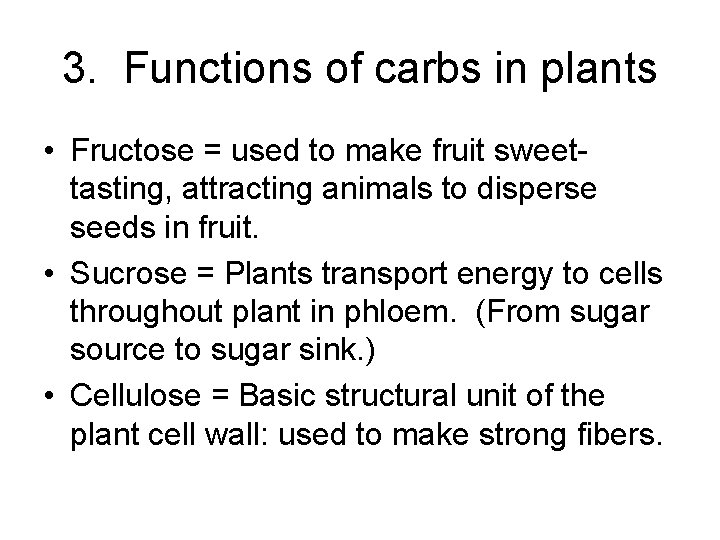
3. Functions of carbs in plants • Fructose = used to make fruit sweettasting, attracting animals to disperse seeds in fruit. • Sucrose = Plants transport energy to cells throughout plant in phloem. (From sugar source to sugar sink. ) • Cellulose = Basic structural unit of the plant cell wall: used to make strong fibers.
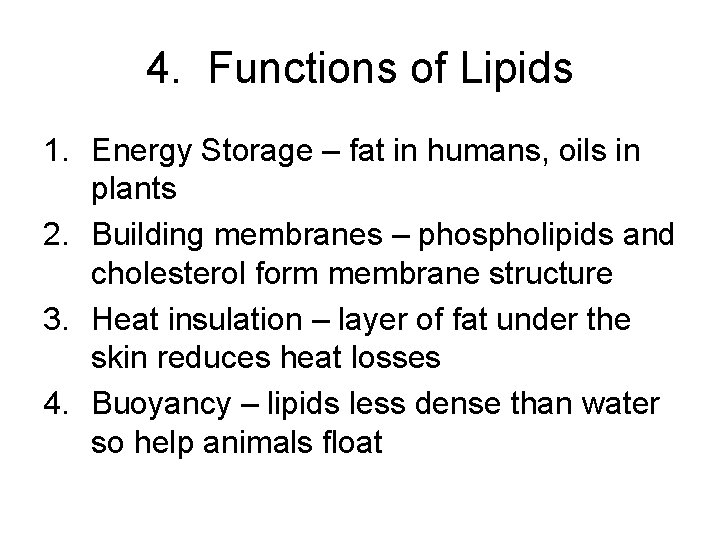
4. Functions of Lipids 1. Energy Storage – fat in humans, oils in plants 2. Building membranes – phospholipids and cholesterol form membrane structure 3. Heat insulation – layer of fat under the skin reduces heat losses 4. Buoyancy – lipids less dense than water so help animals float
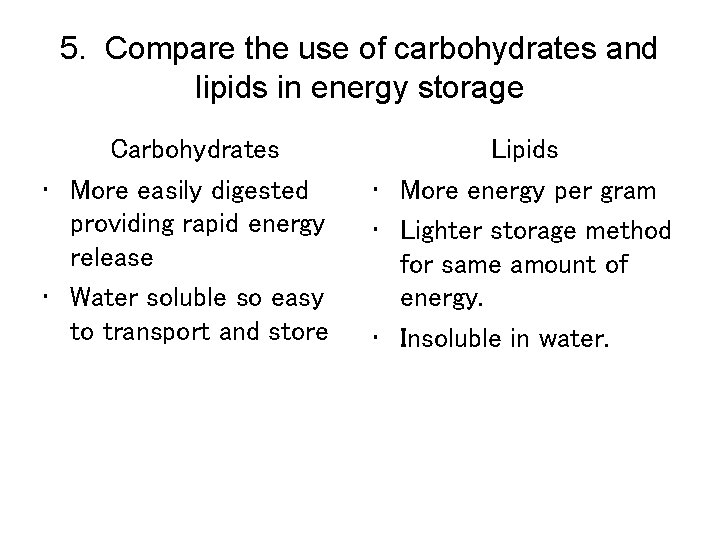
5. Compare the use of carbohydrates and lipids in energy storage Carbohydrates • More easily digested providing rapid energy release • Water soluble so easy to transport and store Lipids • More energy per gram • Lighter storage method for same amount of energy. • Insoluble in water.
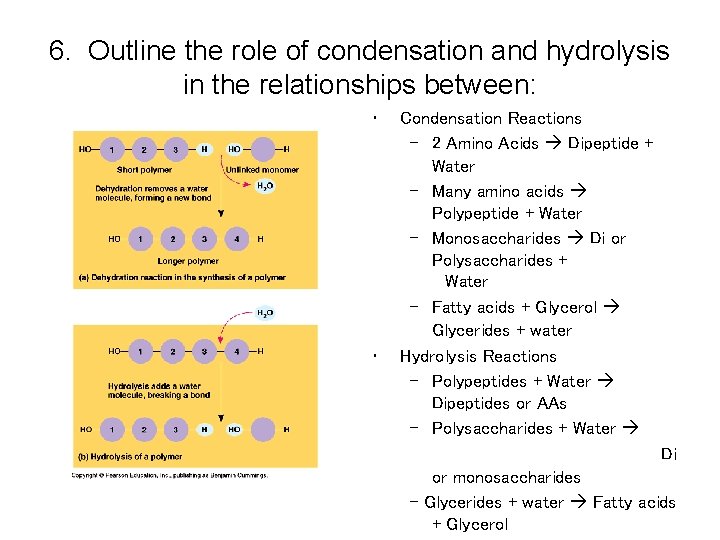
6. Outline the role of condensation and hydrolysis in the relationships between: • • Condensation Reactions – 2 Amino Acids Dipeptide + Water – Many amino acids Polypeptide + Water – Monosaccharides Di or Polysaccharides + Water – Fatty acids + Glycerol Glycerides + water Hydrolysis Reactions – Polypeptides + Water Dipeptides or AAs – Polysaccharides + Water Di or monosaccharides - Glycerides + water Fatty acids + Glycerol
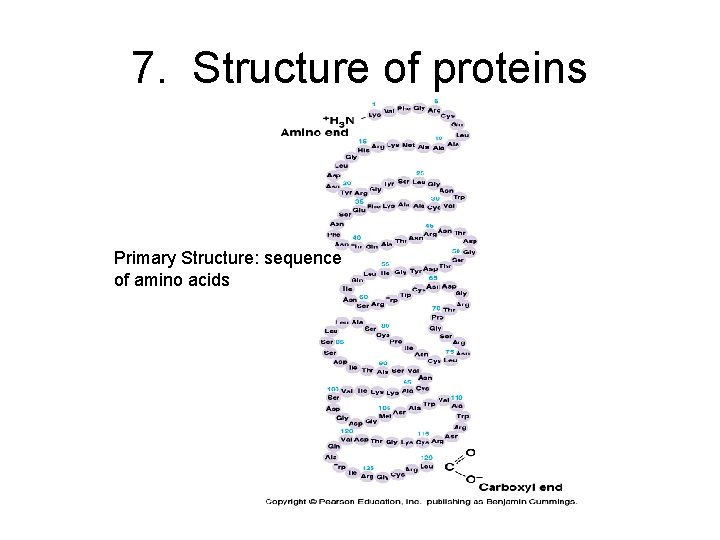
7. Structure of proteins Primary Structure: sequence of amino acids
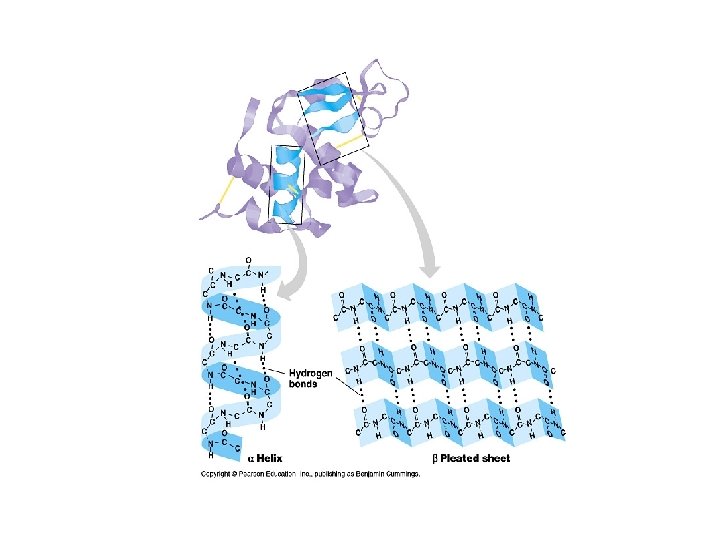
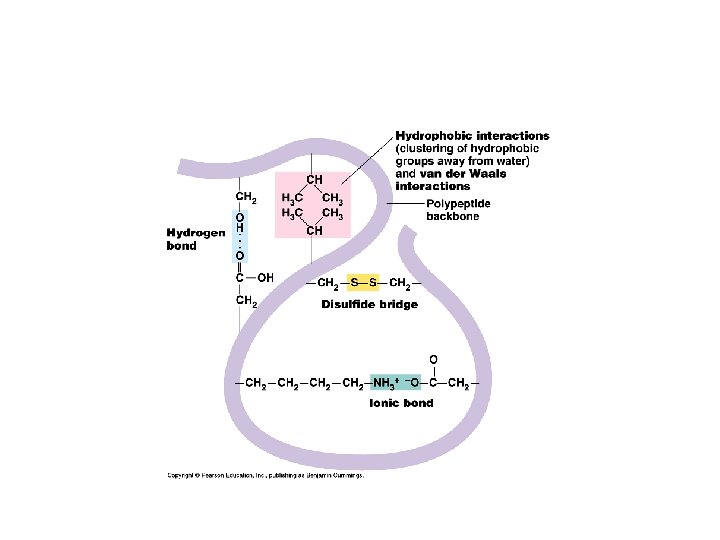
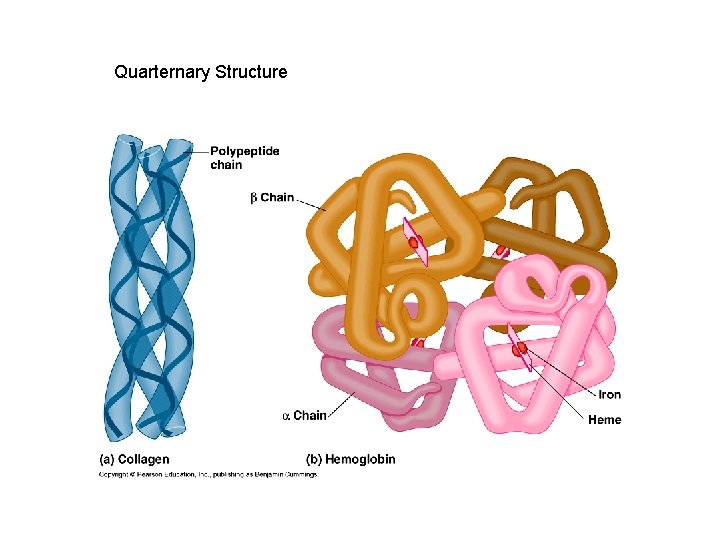
Quarternary Structure
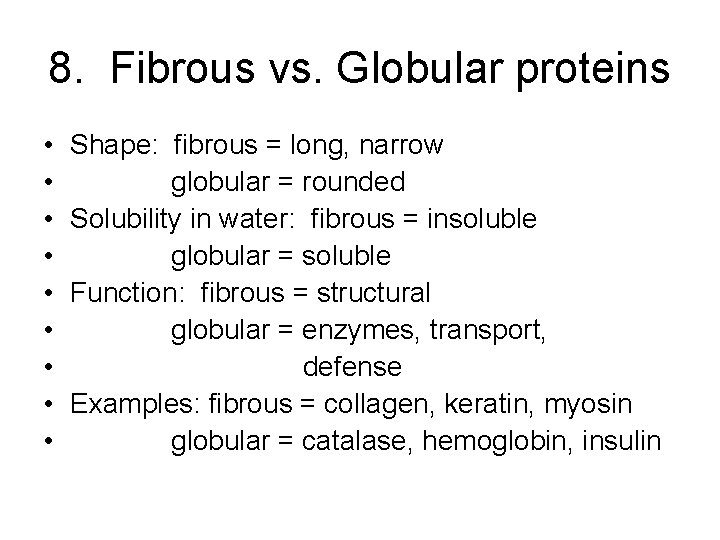
8. Fibrous vs. Globular proteins • • • Shape: fibrous = long, narrow globular = rounded Solubility in water: fibrous = insoluble globular = soluble Function: fibrous = structural globular = enzymes, transport, defense Examples: fibrous = collagen, keratin, myosin globular = catalase, hemoglobin, insulin
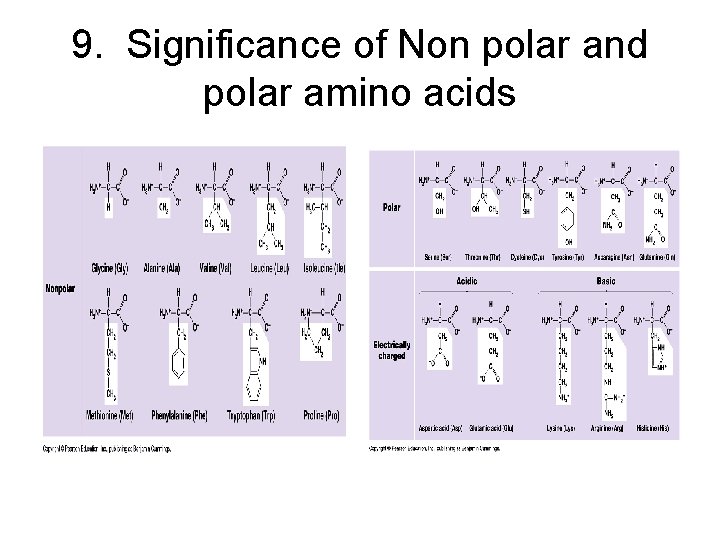
9. Significance of Non polar and polar amino acids
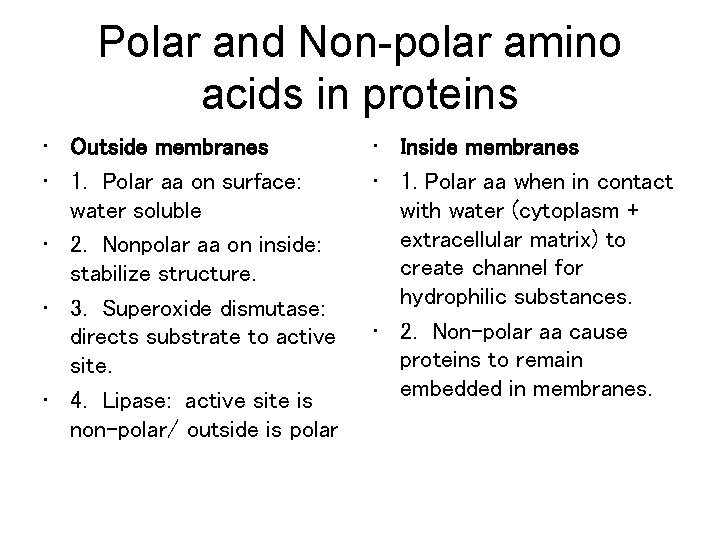
Polar and Non-polar amino acids in proteins • Outside membranes • 1. Polar aa on surface: water soluble • 2. Nonpolar aa on inside: stabilize structure. • 3. Superoxide dismutase: directs substrate to active site. • 4. Lipase: active site is non-polar/ outside is polar • Inside membranes • 1. Polar aa when in contact with water (cytoplasm + extracellular matrix) to create channel for hydrophilic substances. • 2. Non-polar aa cause proteins to remain embedded in membranes.
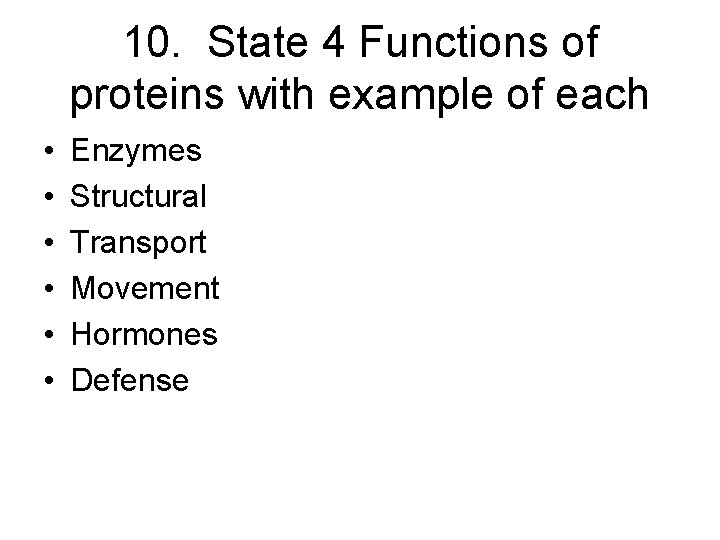
10. State 4 Functions of proteins with example of each • • • Enzymes Structural Transport Movement Hormones Defense
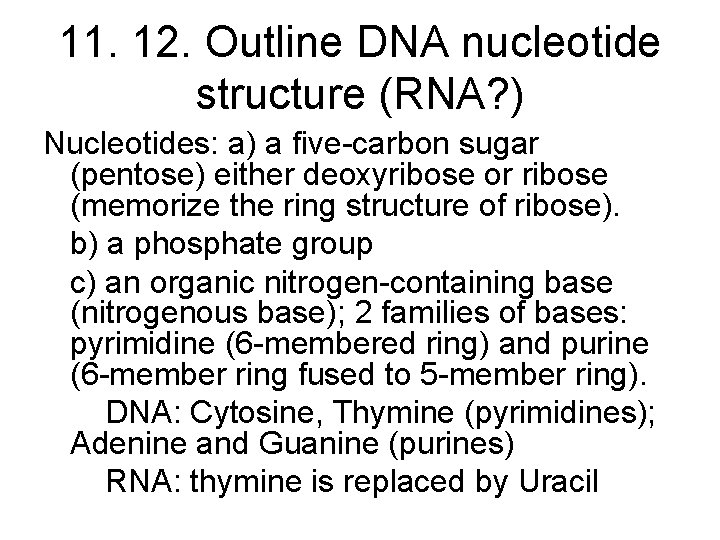
11. 12. Outline DNA nucleotide structure (RNA? ) Nucleotides: a) a five-carbon sugar (pentose) either deoxyribose or ribose (memorize the ring structure of ribose). b) a phosphate group c) an organic nitrogen-containing base (nitrogenous base); 2 families of bases: pyrimidine (6 -membered ring) and purine (6 -member ring fused to 5 -member ring). DNA: Cytosine, Thymine (pyrimidines); Adenine and Guanine (purines) RNA: thymine is replaced by Uracil
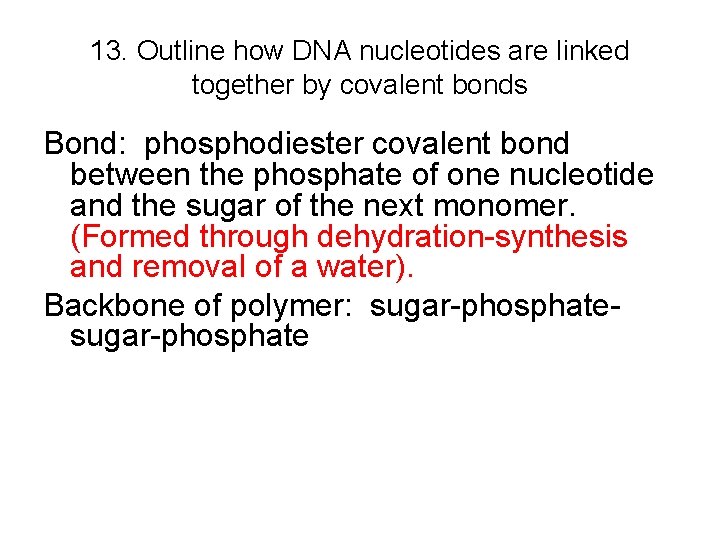
13. Outline how DNA nucleotides are linked together by covalent bonds Bond: phosphodiester covalent bond between the phosphate of one nucleotide and the sugar of the next monomer. (Formed through dehydration-synthesis and removal of a water). Backbone of polymer: sugar-phosphate
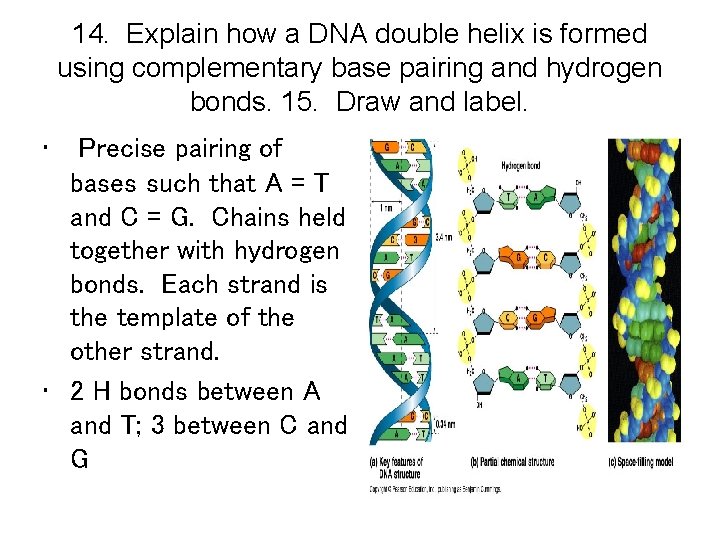
14. Explain how a DNA double helix is formed using complementary base pairing and hydrogen bonds. 15. Draw and label. • Precise pairing of bases such that A = T and C = G. Chains held together with hydrogen bonds. Each strand is the template of the other strand. • 2 H bonds between A and T; 3 between C and G
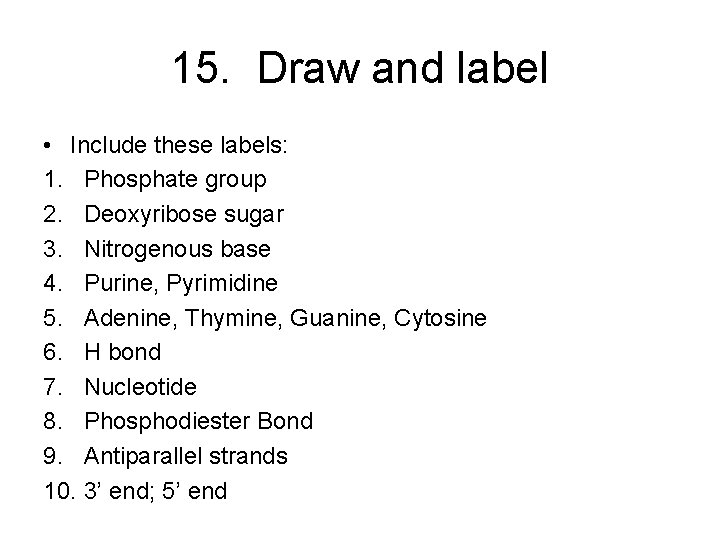
15. Draw and label • Include these labels: 1. Phosphate group 2. Deoxyribose sugar 3. Nitrogenous base 4. Purine, Pyrimidine 5. Adenine, Thymine, Guanine, Cytosine 6. H bond 7. Nucleotide 8. Phosphodiester Bond 9. Antiparallel strands 10. 3’ end; 5’ end
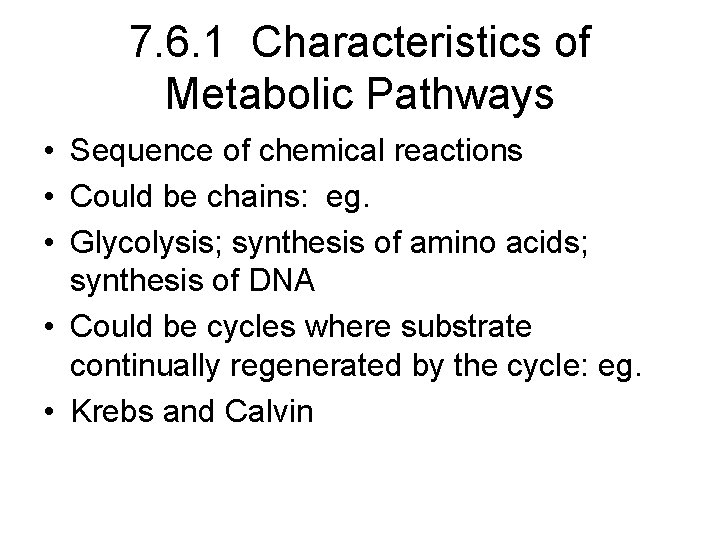
7. 6. 1 Characteristics of Metabolic Pathways • Sequence of chemical reactions • Could be chains: eg. • Glycolysis; synthesis of amino acids; synthesis of DNA • Could be cycles where substrate continually regenerated by the cycle: eg. • Krebs and Calvin
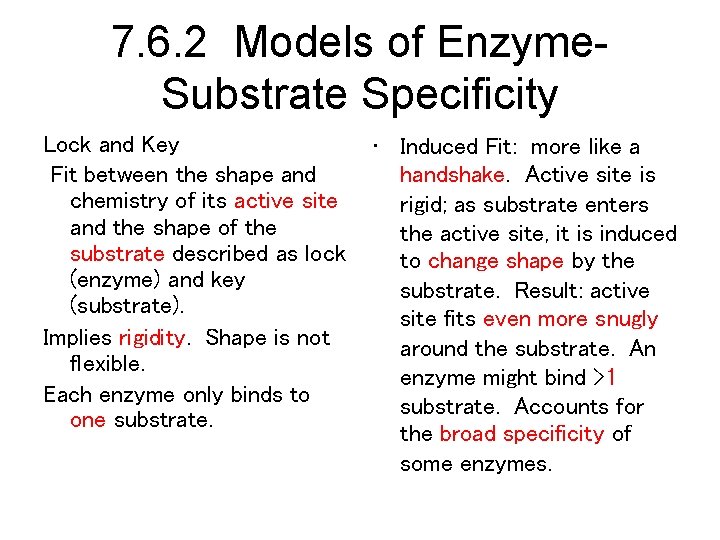
7. 6. 2 Models of Enzyme. Substrate Specificity Lock and Key Fit between the shape and chemistry of its active site and the shape of the substrate described as lock (enzyme) and key (substrate). Implies rigidity. Shape is not flexible. Each enzyme only binds to one substrate. • Induced Fit: more like a handshake. Active site is rigid; as substrate enters the active site, it is induced to change shape by the substrate. Result: active site fits even more snugly around the substrate. An enzyme might bind >1 substrate. Accounts for the broad specificity of some enzymes.
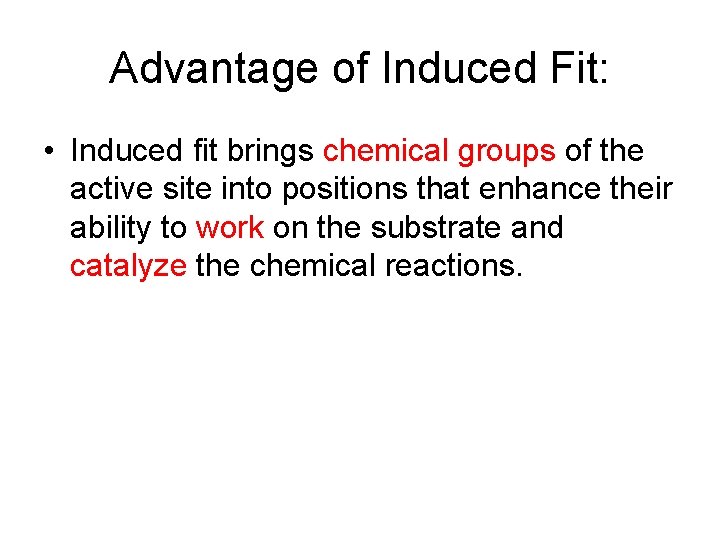
Advantage of Induced Fit: • Induced fit brings chemical groups of the active site into positions that enhance their ability to work on the substrate and catalyze the chemical reactions.
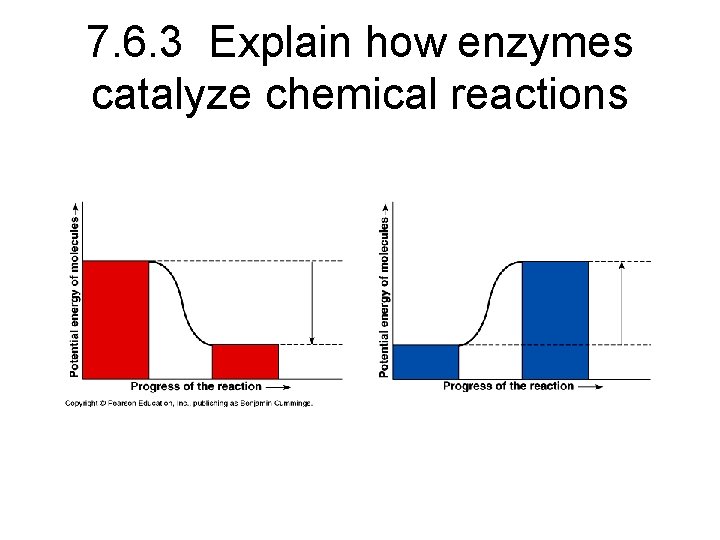
7. 6. 3 Explain how enzymes catalyze chemical reactions
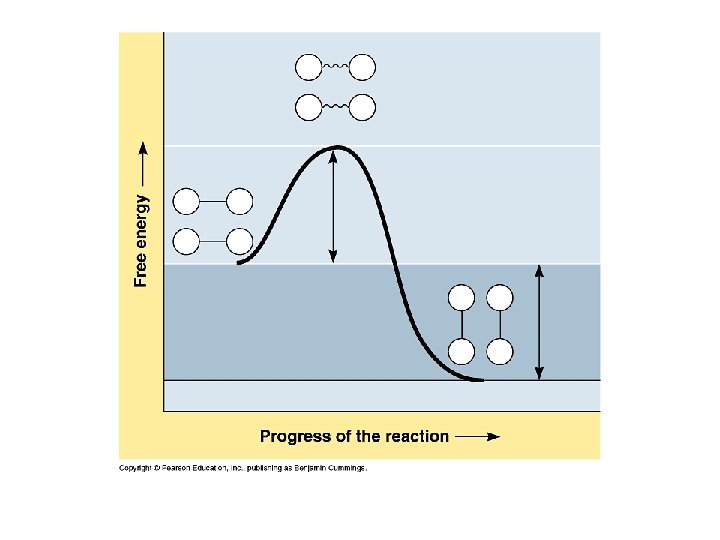
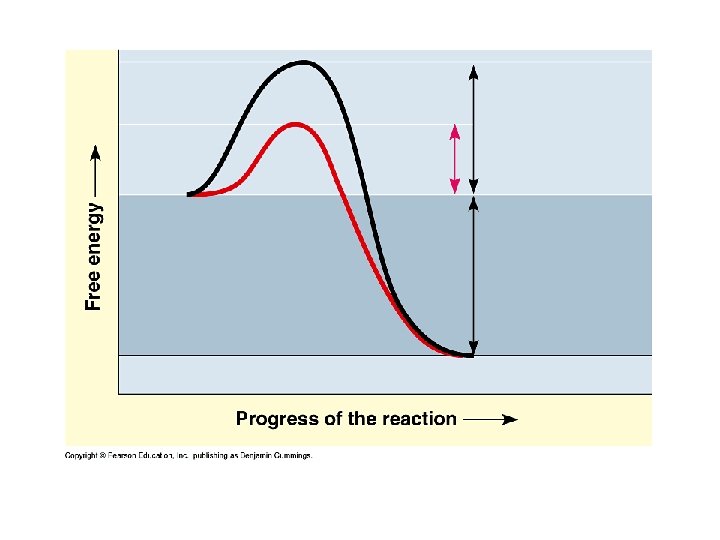
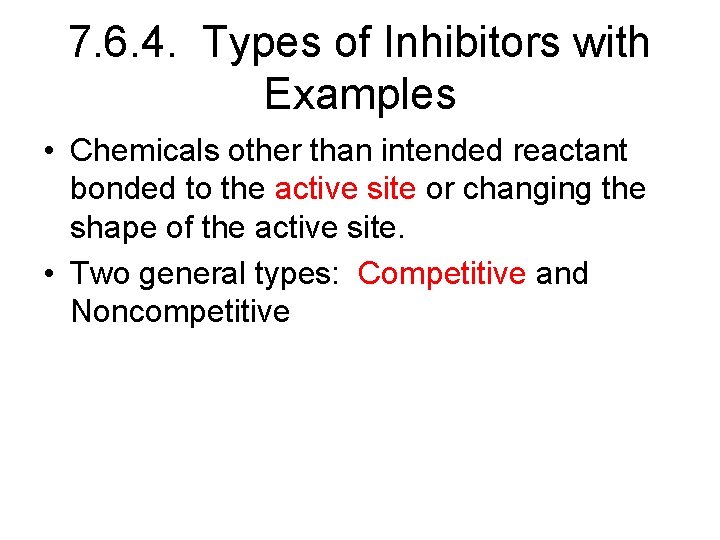
7. 6. 4. Types of Inhibitors with Examples • Chemicals other than intended reactant bonded to the active site or changing the shape of the active site. • Two general types: Competitive and Noncompetitive
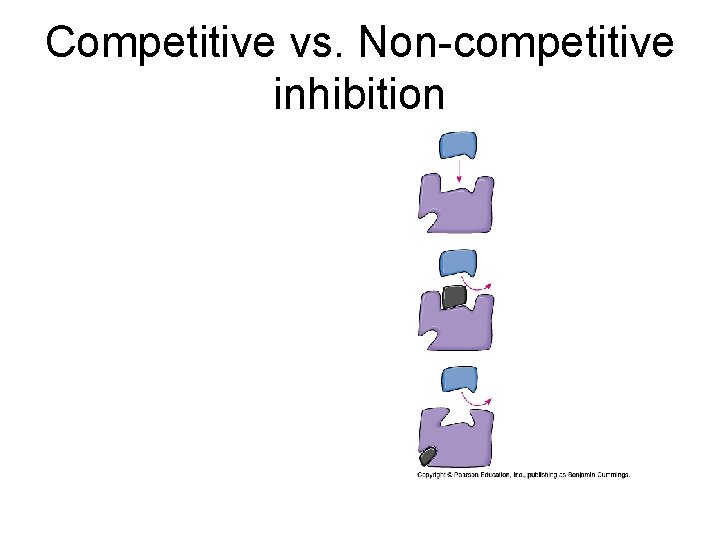
Competitive vs. Non-competitive inhibition
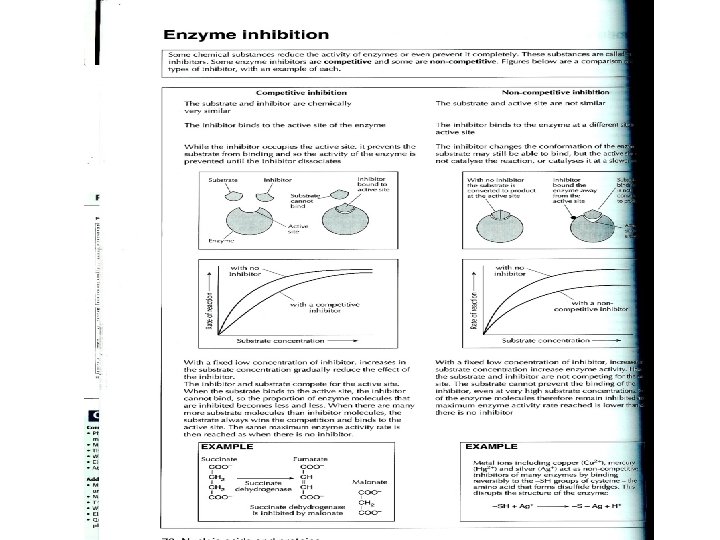
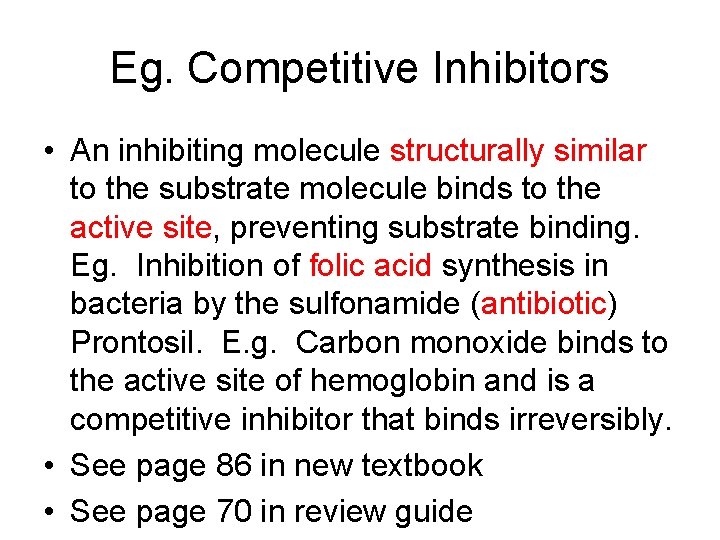
Eg. Competitive Inhibitors • An inhibiting molecule structurally similar to the substrate molecule binds to the active site, preventing substrate binding. Eg. Inhibition of folic acid synthesis in bacteria by the sulfonamide (antibiotic) Prontosil. E. g. Carbon monoxide binds to the active site of hemoglobin and is a competitive inhibitor that binds irreversibly. • See page 86 in new textbook • See page 70 in review guide
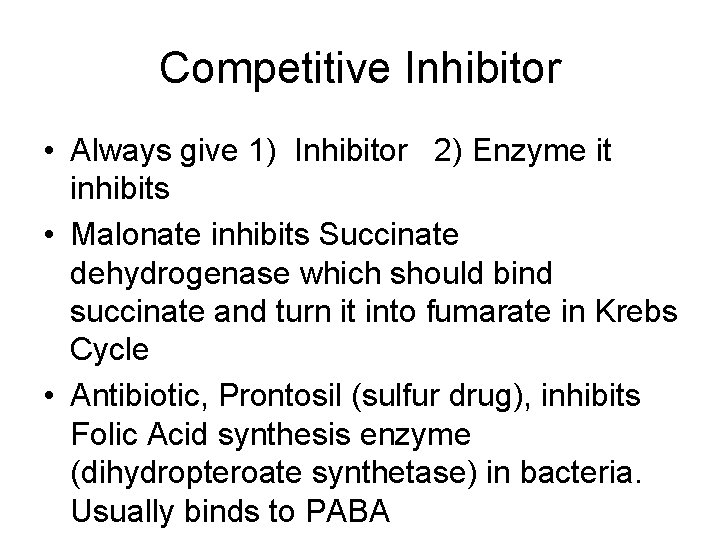
Competitive Inhibitor • Always give 1) Inhibitor 2) Enzyme it inhibits • Malonate inhibits Succinate dehydrogenase which should bind succinate and turn it into fumarate in Krebs Cycle • Antibiotic, Prontosil (sulfur drug), inhibits Folic Acid synthesis enzyme (dihydropteroate synthetase) in bacteria. Usually binds to PABA
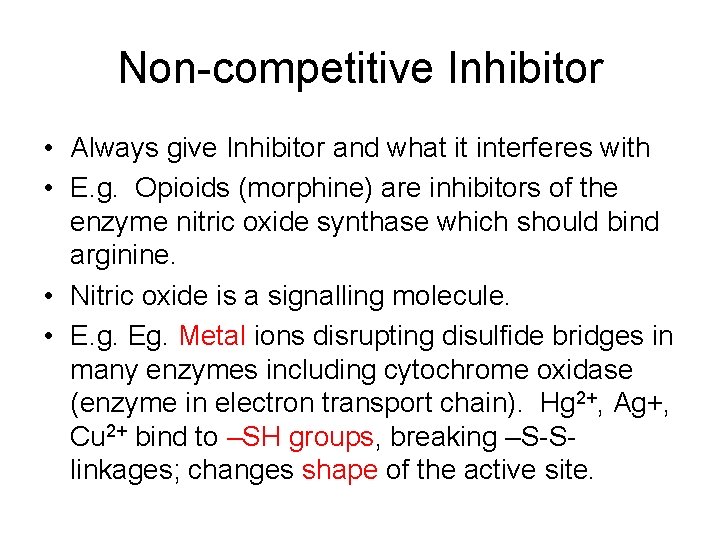
Non-competitive Inhibitor • Always give Inhibitor and what it interferes with • E. g. Opioids (morphine) are inhibitors of the enzyme nitric oxide synthase which should bind arginine. • Nitric oxide is a signalling molecule. • E. g. Eg. Metal ions disrupting disulfide bridges in many enzymes including cytochrome oxidase (enzyme in electron transport chain). Hg 2+, Ag+, Cu 2+ bind to –SH groups, breaking –S-Slinkages; changes shape of the active site.
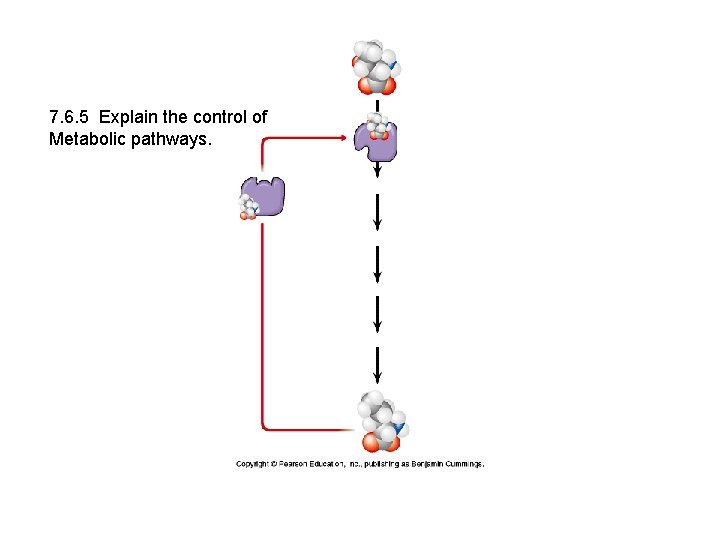
7. 6. 5 Explain the control of Metabolic pathways.
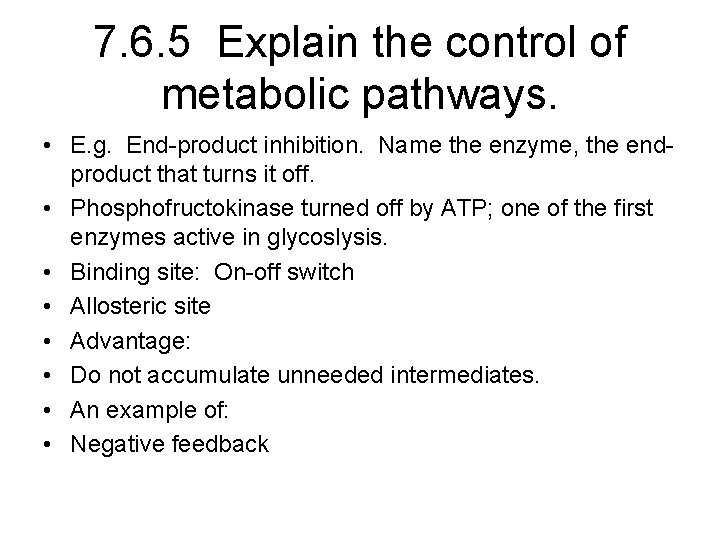
7. 6. 5 Explain the control of metabolic pathways. • E. g. End-product inhibition. Name the enzyme, the endproduct that turns it off. • Phosphofructokinase turned off by ATP; one of the first enzymes active in glycoslysis. • Binding site: On-off switch • Allosteric site • Advantage: • Do not accumulate unneeded intermediates. • An example of: • Negative feedback
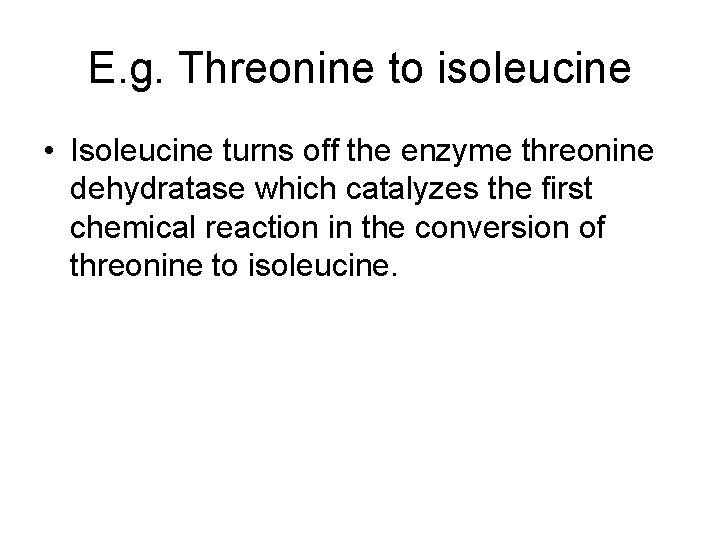
E. g. Threonine to isoleucine • Isoleucine turns off the enzyme threonine dehydratase which catalyzes the first chemical reaction in the conversion of threonine to isoleucine.
Express 602200 in scientific notation.
97 700 000 000 000 000 000 000 in scientific notation
71 000 in scientific notation
100 100 100 100 100
Percent greater than 100 and less than 1
Alphablocks
Fractions greater less than or equal to
Compound inequality examples
Greater than key words
It is greater than god and more evil
Odd one out fractions
090-0000-0000
1, 10, 100, 1000, 10000
100 iops/gb and 100,000 iops per volume oci
Organic molecules vs inorganic molecules
The most dangerous game dialectical journal
How does monopoly arise?
Less than equal to graph
When minuend=subtrahend difference=
If mean greater than median
Mother any distance analysis
Delta g greater than 0
The cell is a living unit greater than the sum of its parts
Which animal phylum has greater complexity than urochordata
A cell is a living unit greater than the sum of its parts
Which fraction is greater than 1/2
Grace greater than our sin history
What is greater than 2/3
The verbal phrase of the inequality symbol
Adrenarche
Capsule flagella pili nucleoid desmosome
Type 1 error statistics
Knowledge empowers imagination
Great commission
Monopoly atc curve
Pedipalpos quelados
Milli micro nano
1 600 000 000
140 000 000 in scientific notation
4 500 000 000
Advantages of scientific notation
Frans cooijmans
1,000 x 3,000
450 scientific notation
2,340,000,000
240 000
4 500 000
4 000 000
Auld lang syne lemuria
2340000000
210 in scientific notation
1-000-000-0000
347 000 in scientific notation
Express 4,980,000, 000 in scientific notation
4 500 000
110 000 110 & 111 000 111
100 000 reasons
Never before oh my soul
Roanoke regional loan center
100 000 reasons
A is an odd number which rounds to 100 000
80000 100
Round 683 492 to the nearest hundred thousand
Arizona state savings credit union
This veterans basic entitlement is $36 000*
10 000/100
200+200+300
What's 100 + 100
200+200+100+100
100+100=200
Gcse box plot questions
300 + 300 + 200
200+200+300+300
Malloc lab 100/100
Romanos hasta 1000
C/100=f-32/180=k-273/100
240 sayısının 60'ı kaçtır
Héroïne dans la guerre de 100 ans (100 years war).
106 is prime number
5730x5
Er than more than
Your love is deeper than the ocean higher than the heavens
Lab
Macromolecules
Why are macromolecules important
Macromolecules doodle notes
What are macromolecules
What are macromolecules
Subunits of fat
Which type of macromolecule stores genetic information?
Macromolecules in food lab
What macromolecules make up a macadamia nut
Macromolecules
Macromolecules cheat sheet
Amino acid joke
What are macromolecules
Importance of each biological macromolecules
Macromolecules in cellular respiration
Macromolecules you are what you eat
Macromolecule superhero
Macromolecules in food
How are indicators used to detect macromolecules
Macromolecules