XII Gene Regulation Overview All cells in an
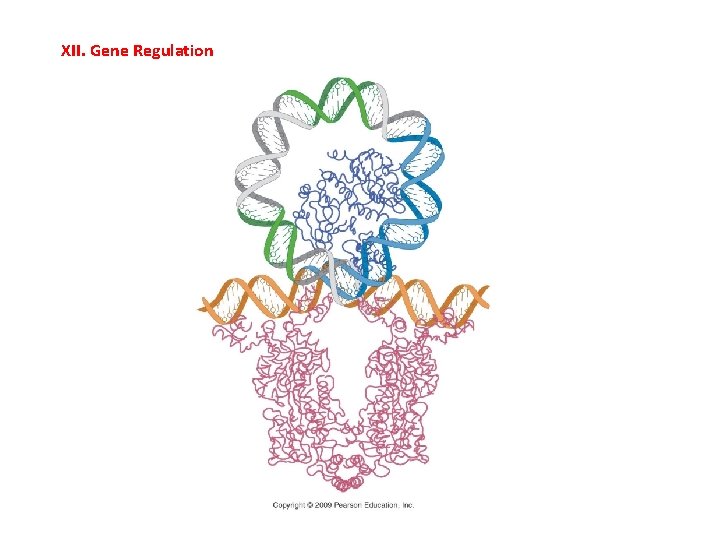
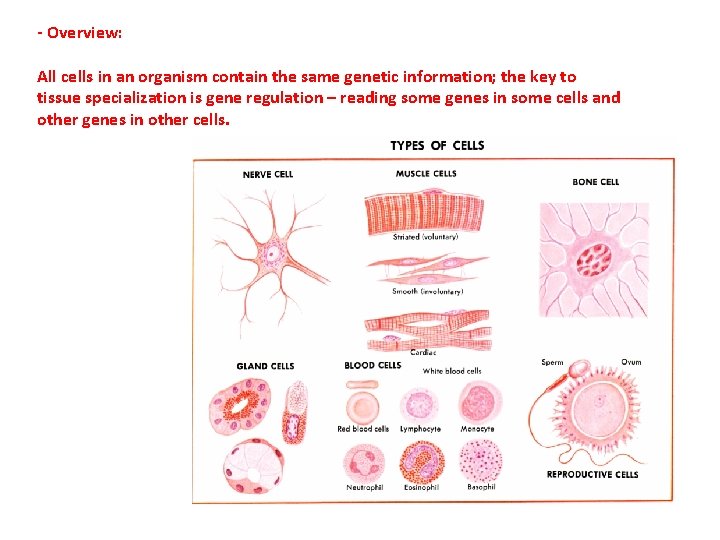
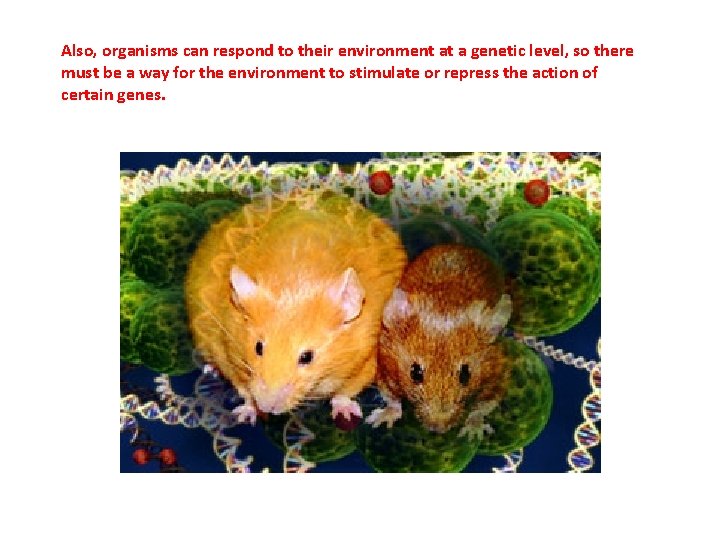
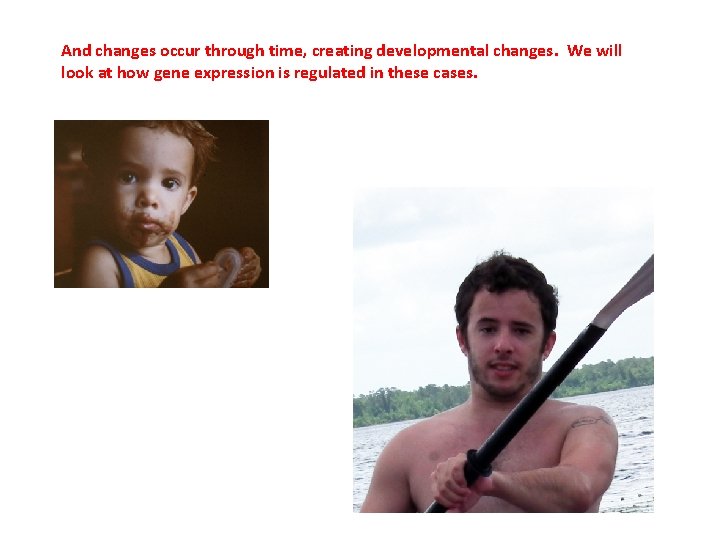
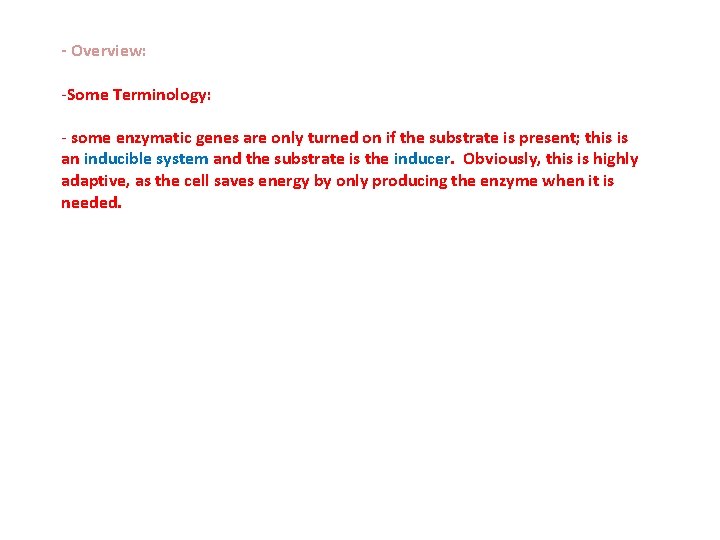
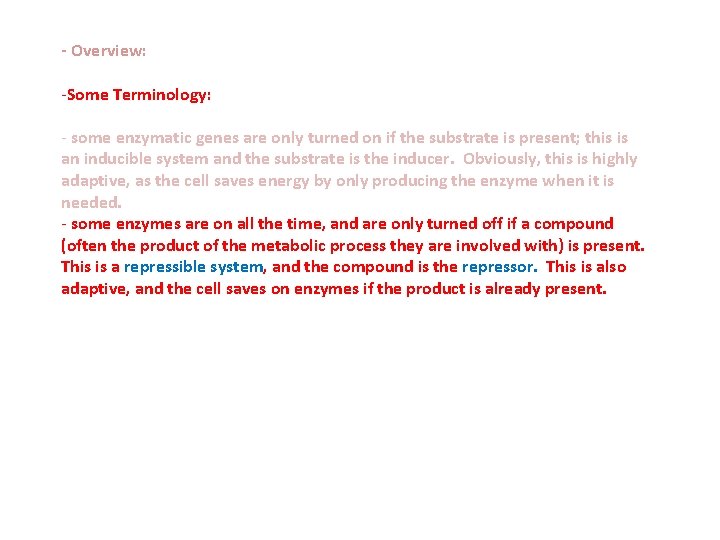
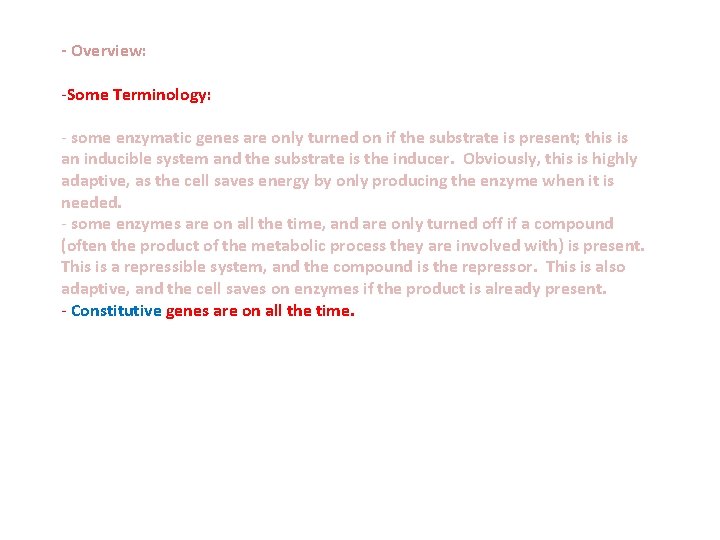
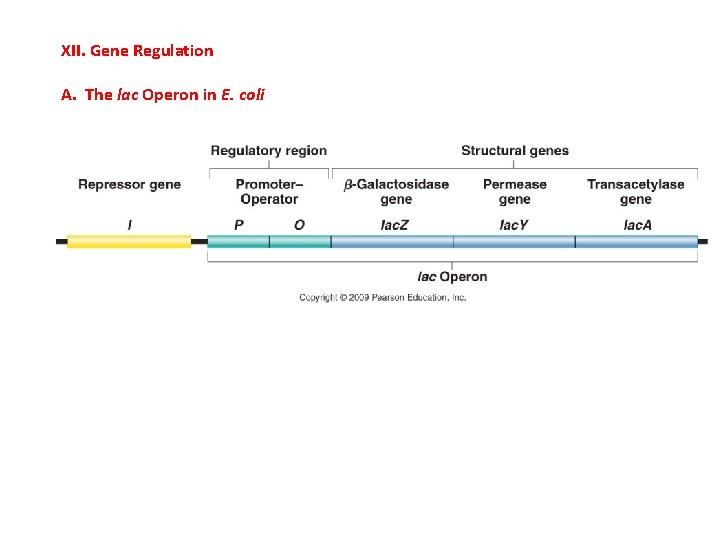
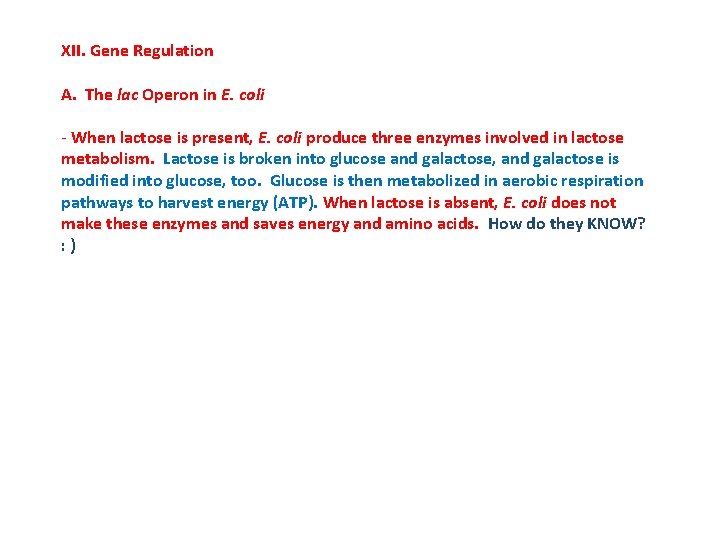
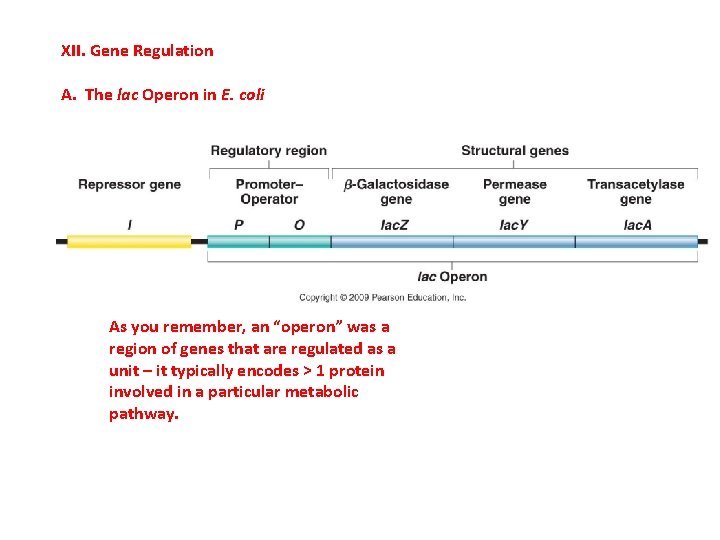
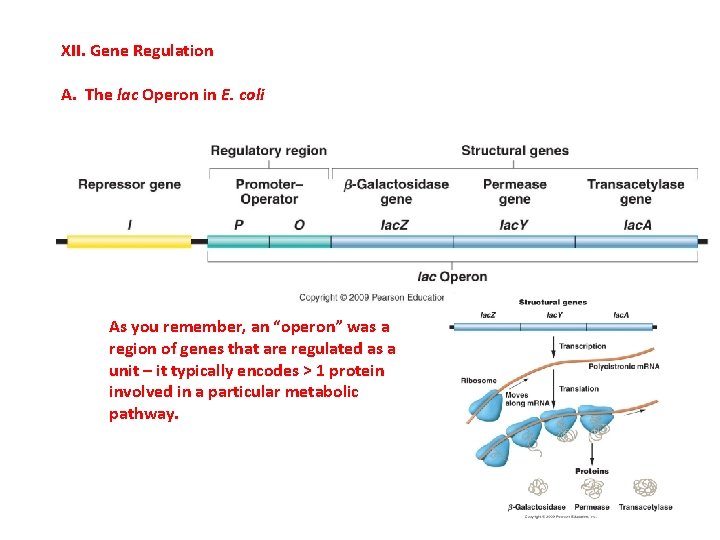
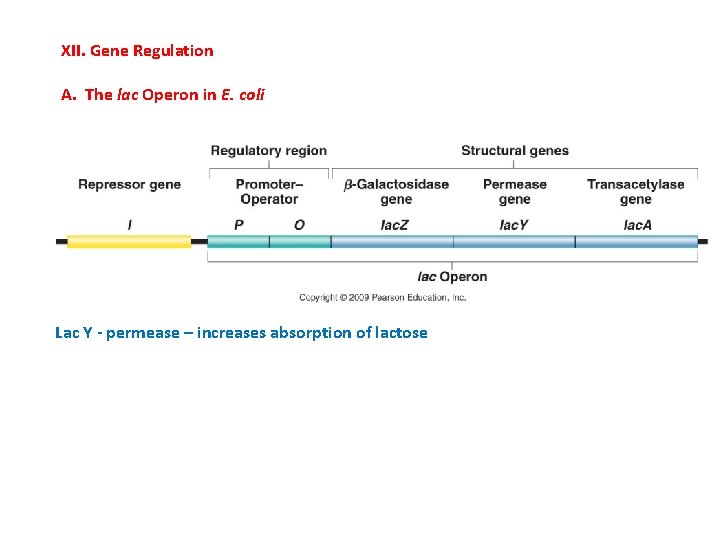
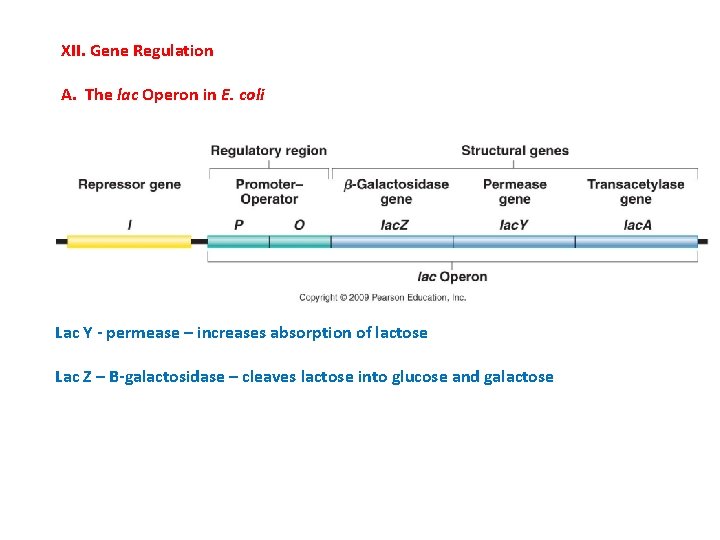
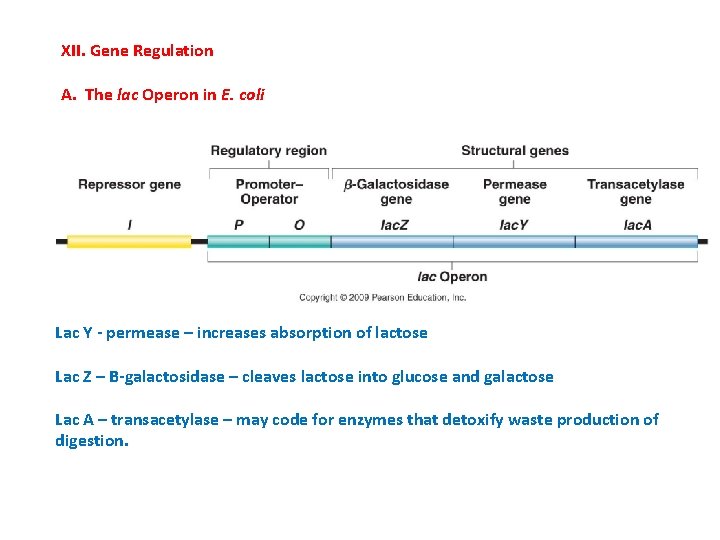
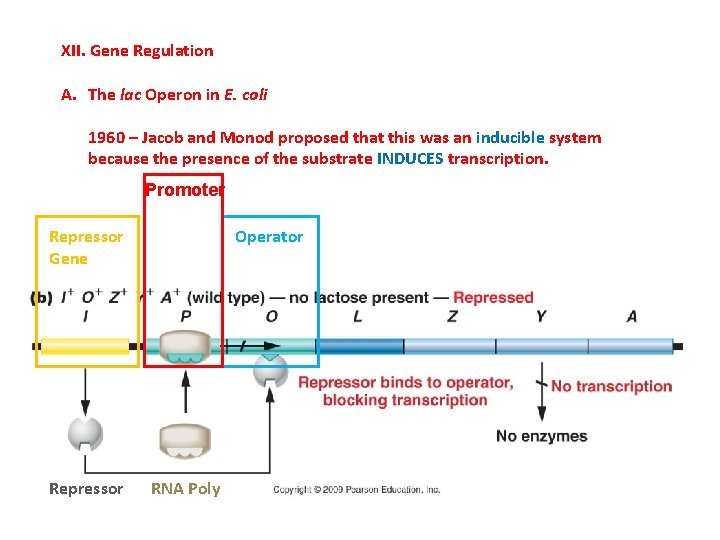
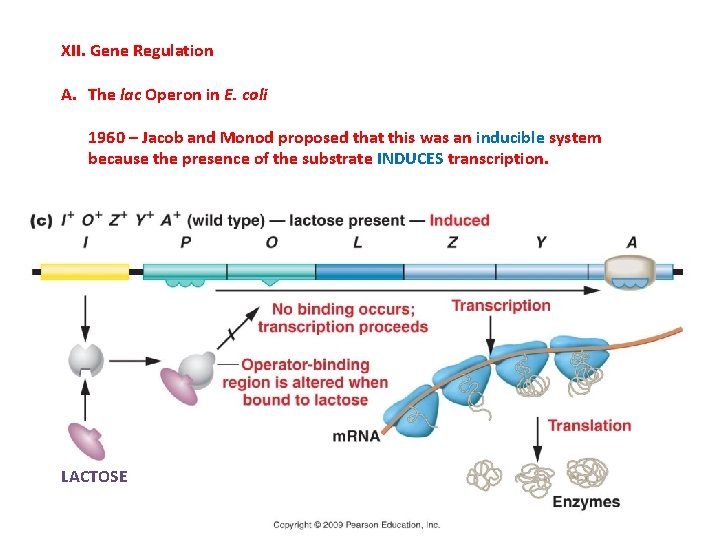
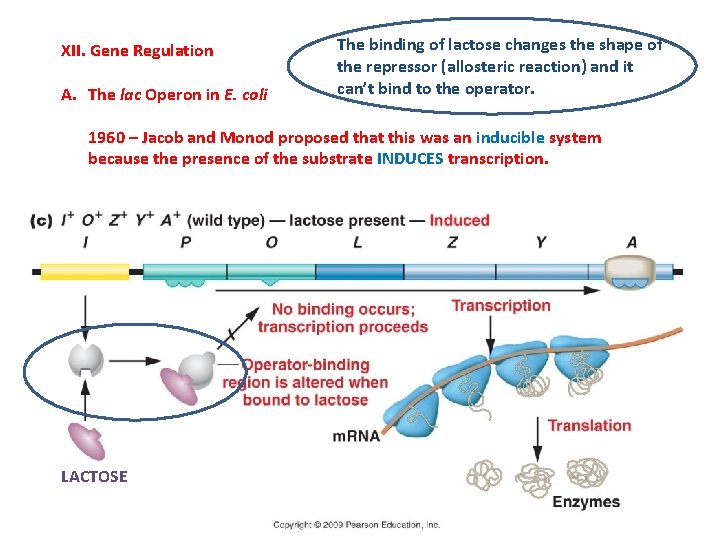
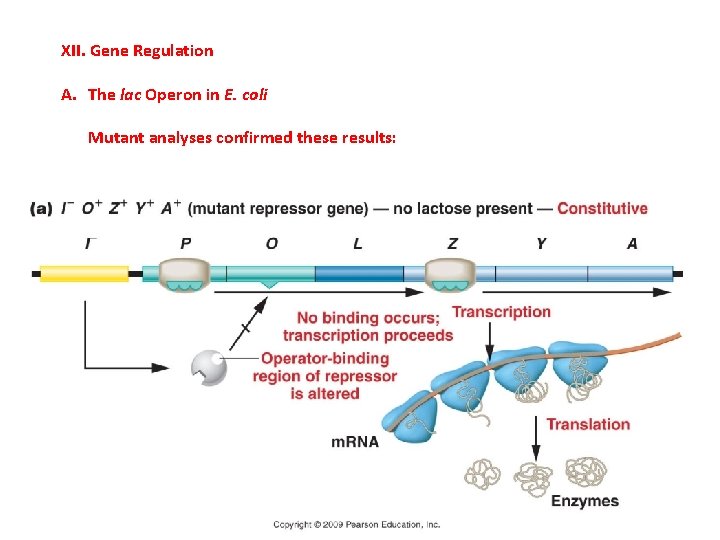
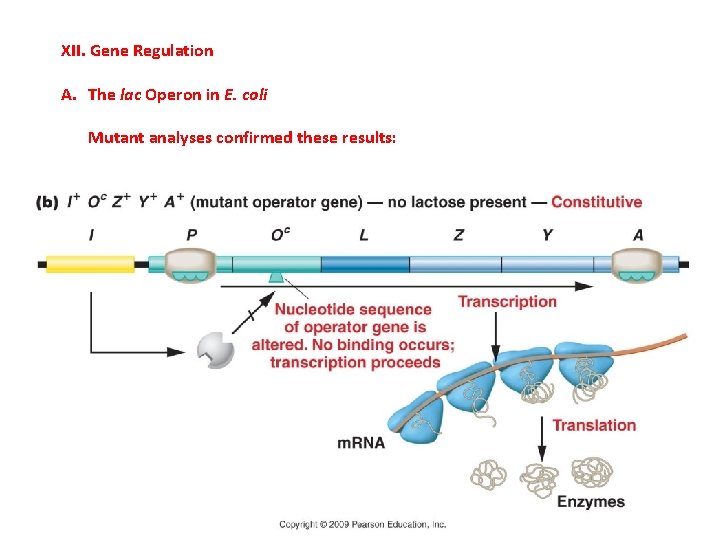
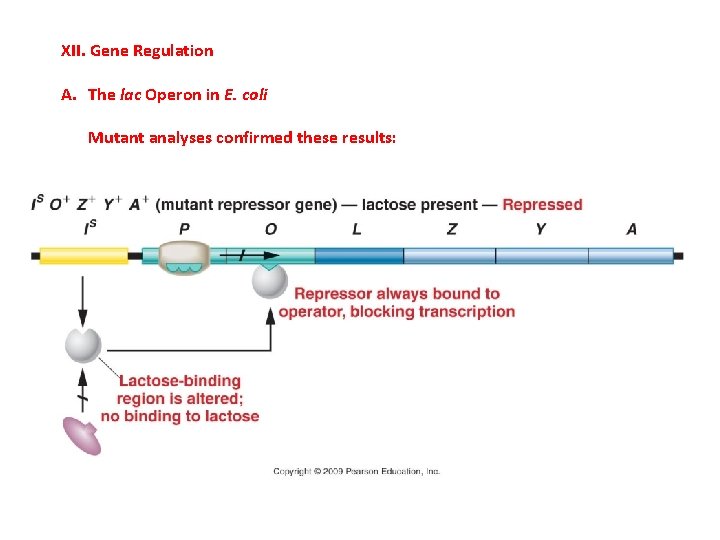
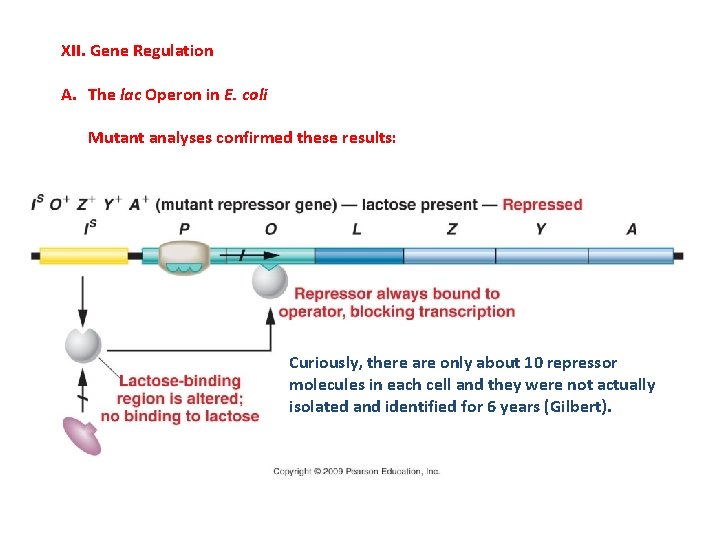
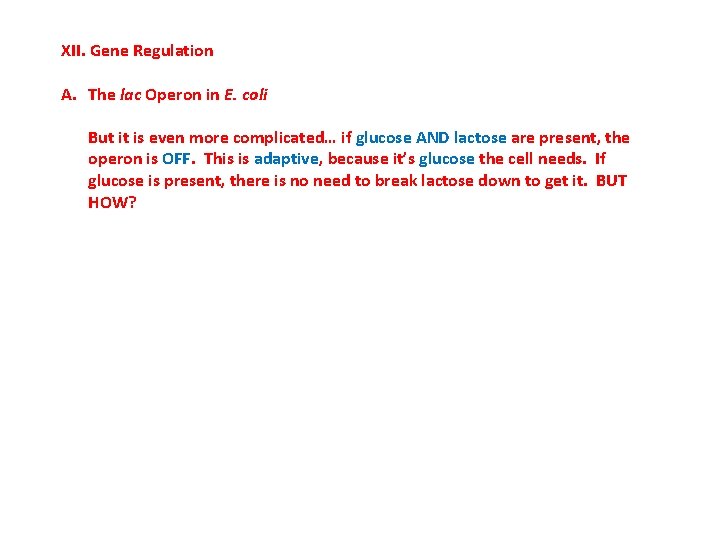
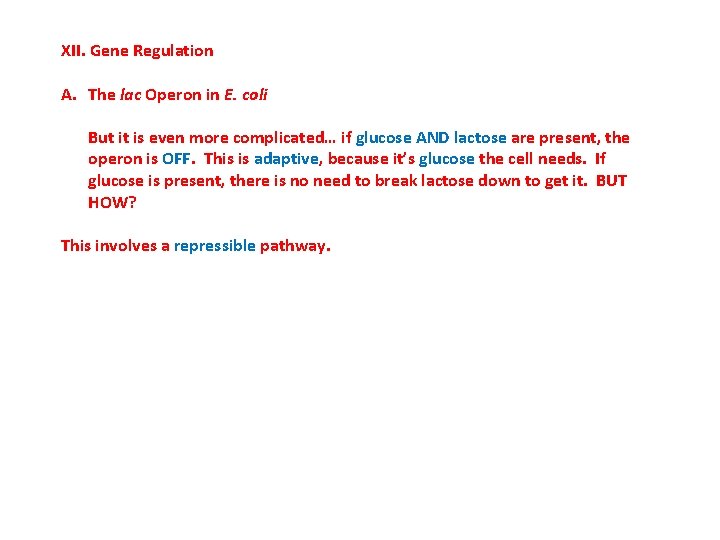
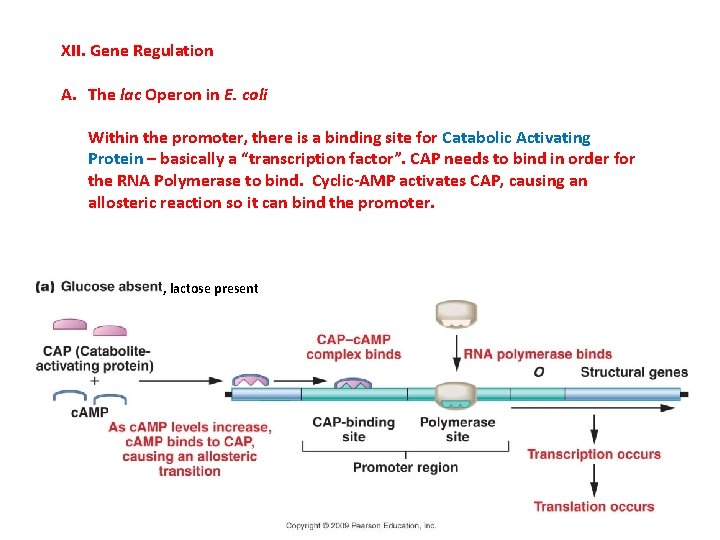
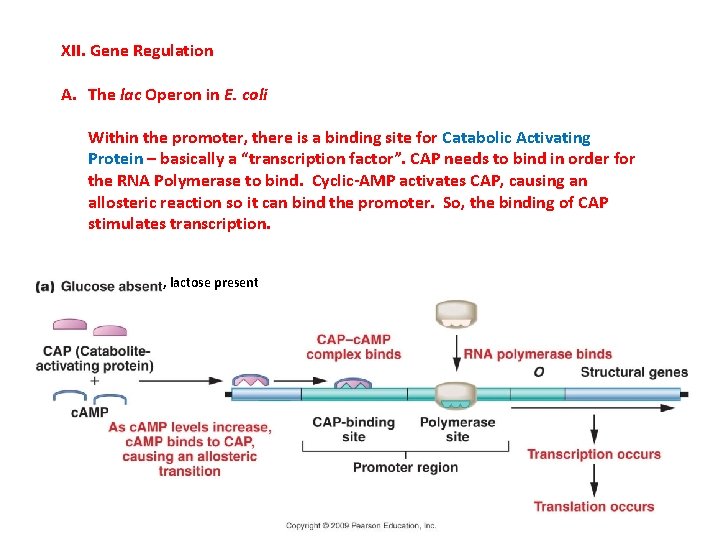
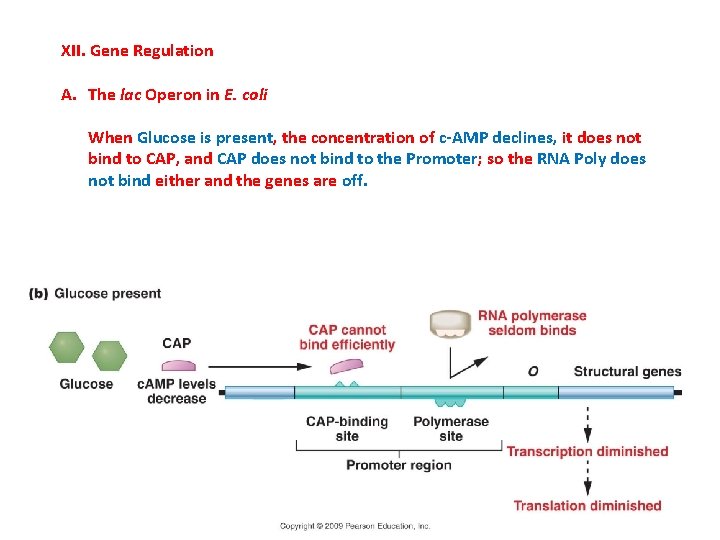
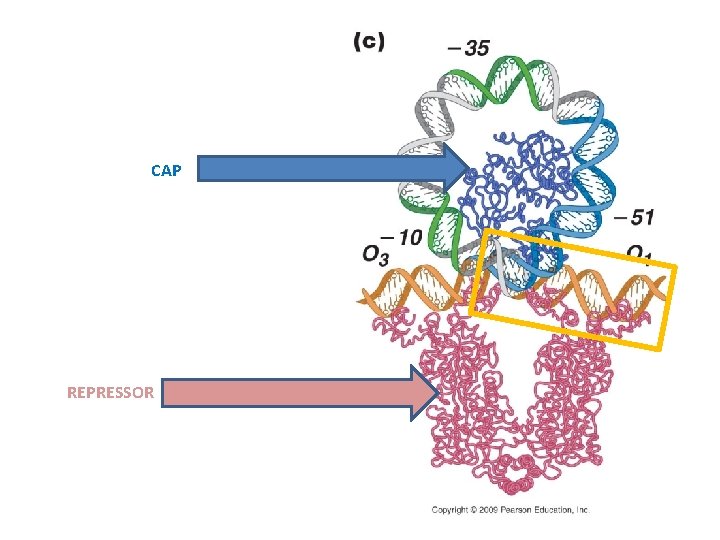
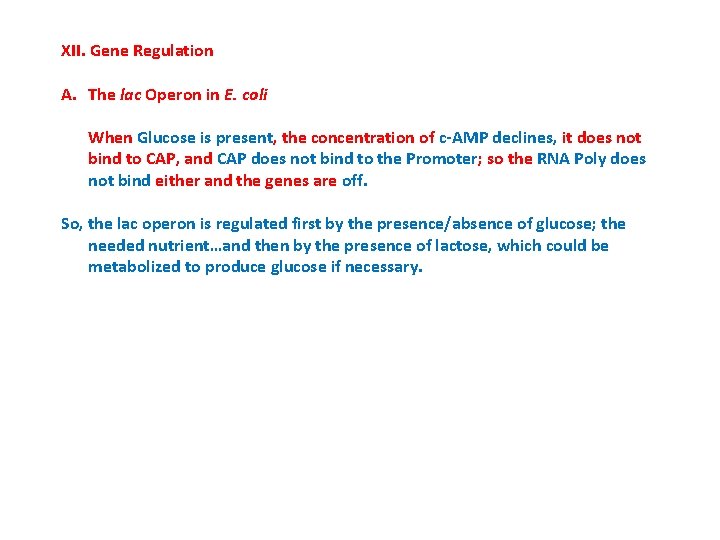
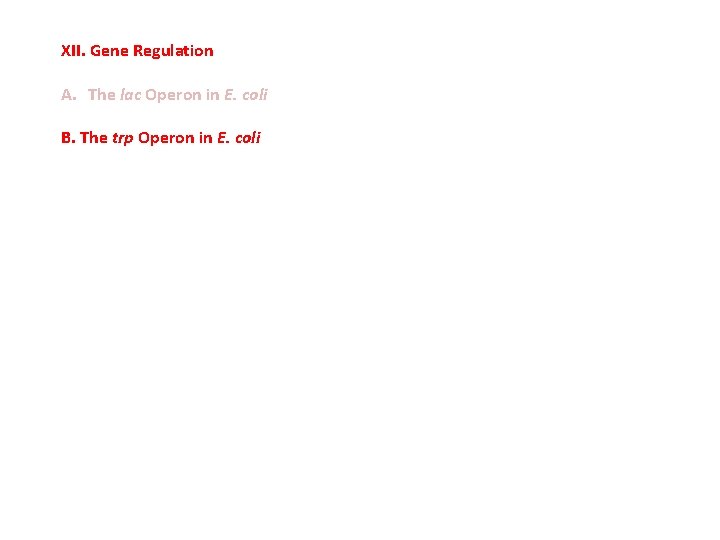
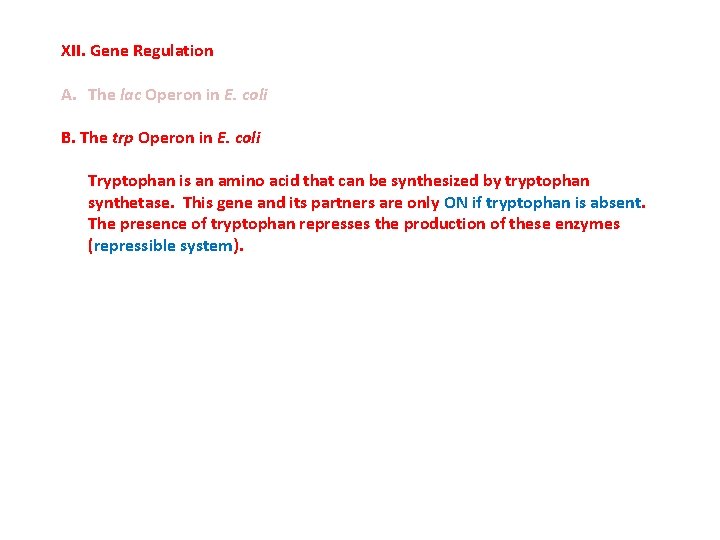
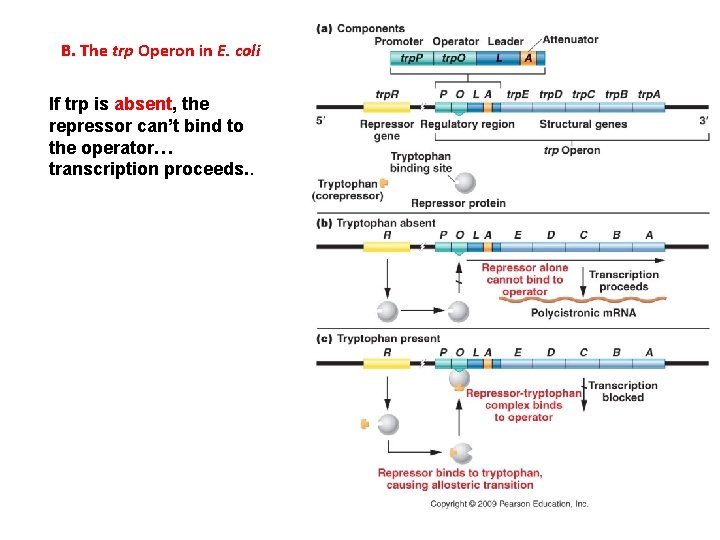
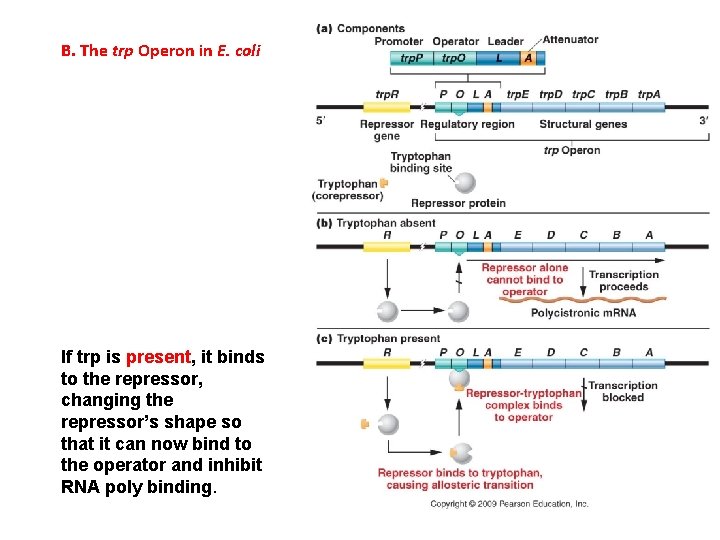
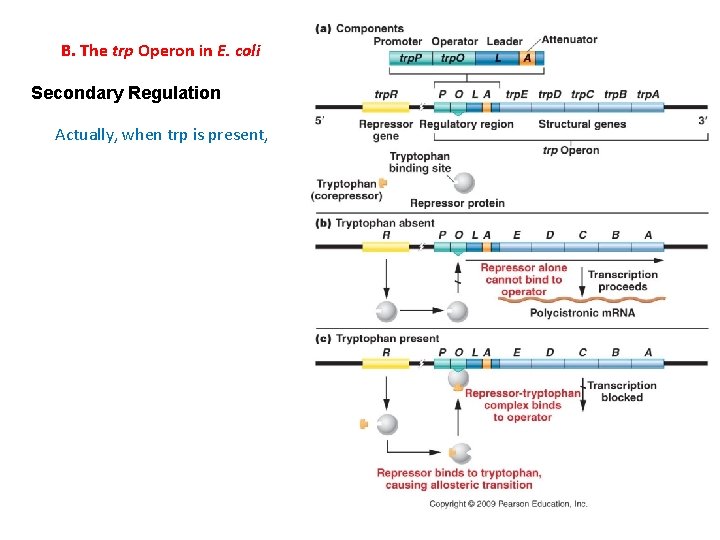
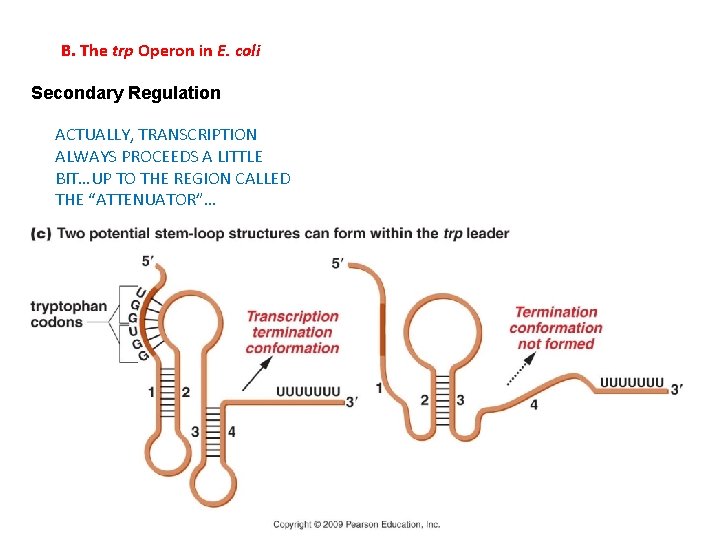
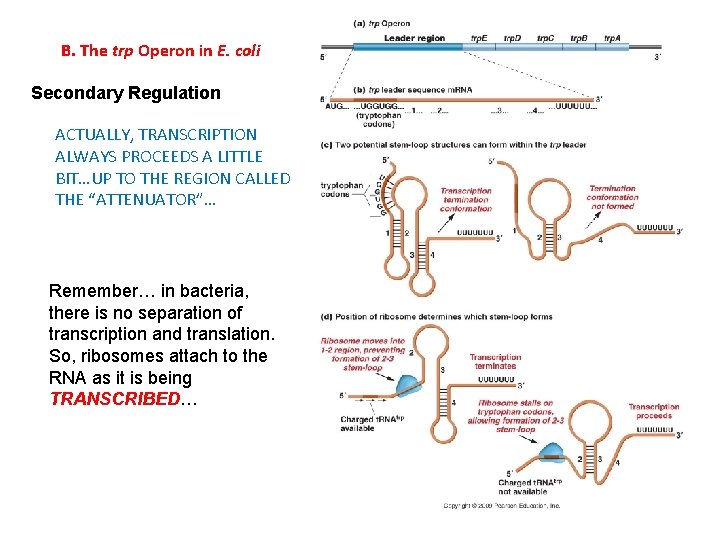
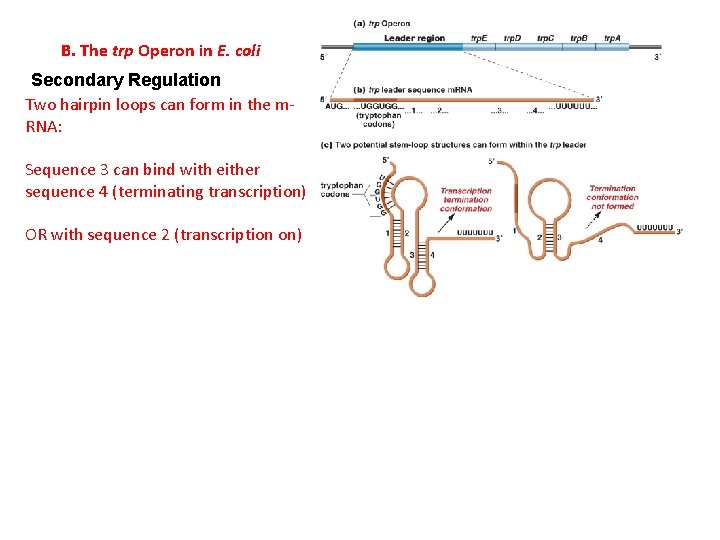
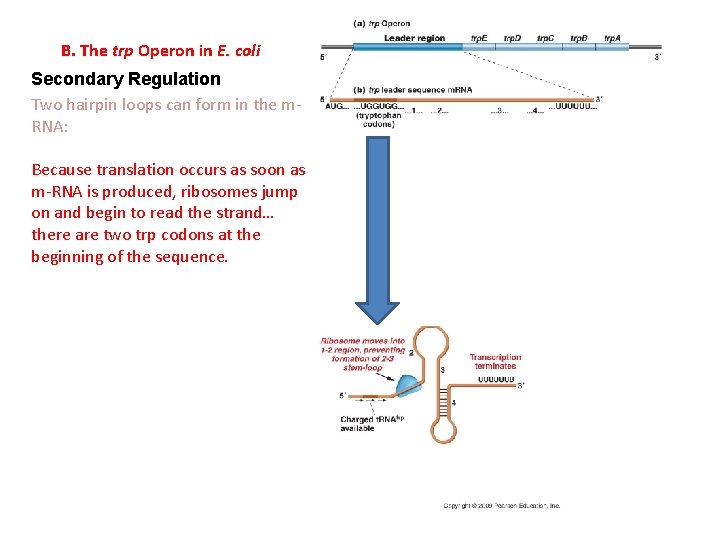
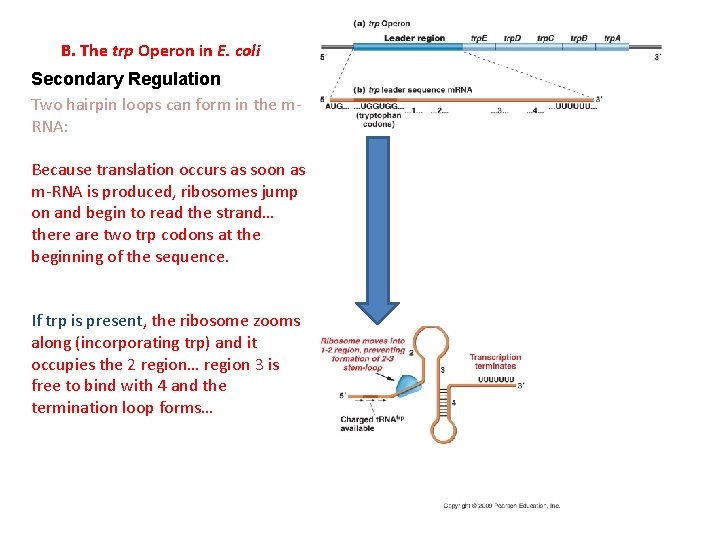
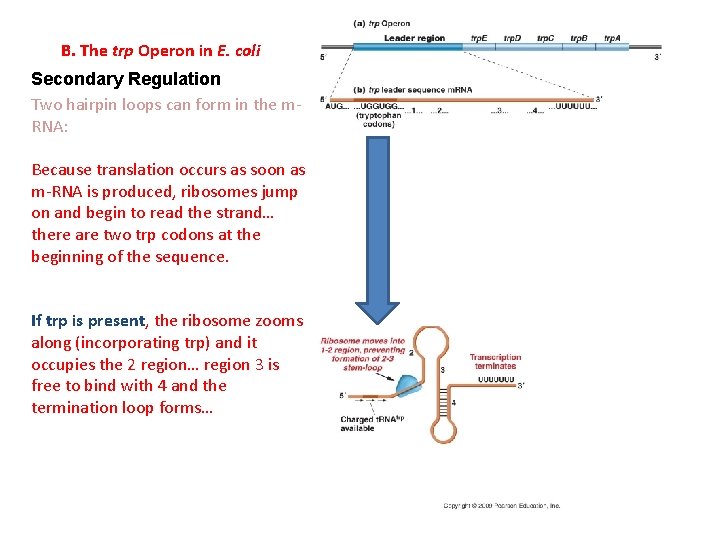
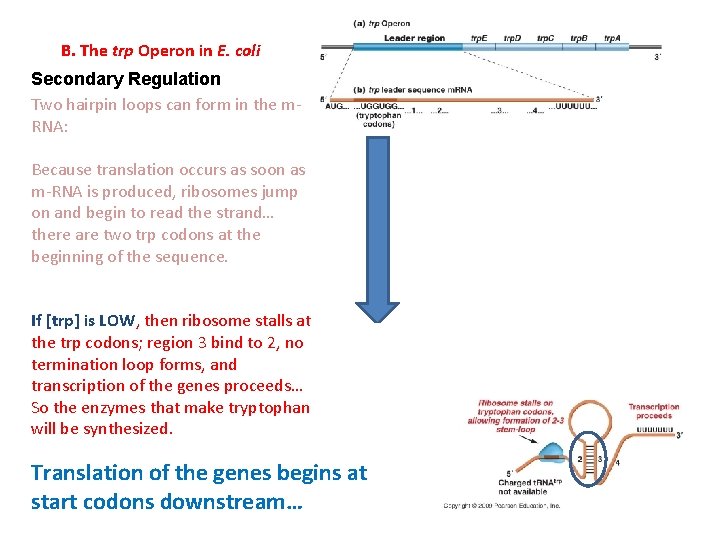
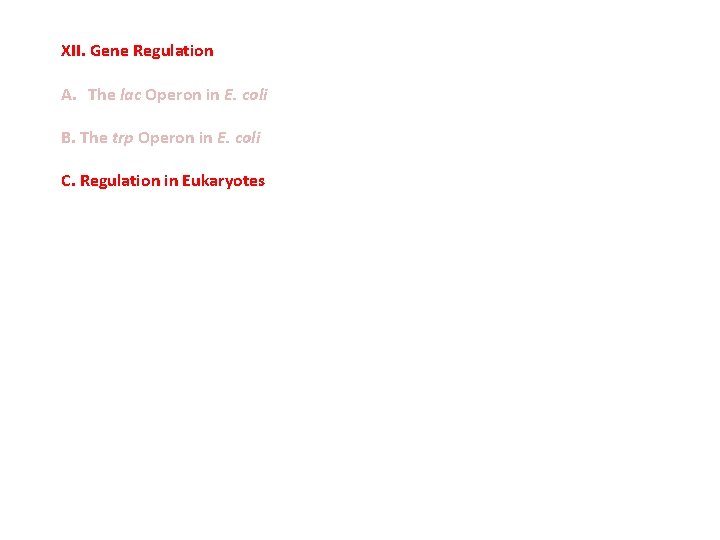
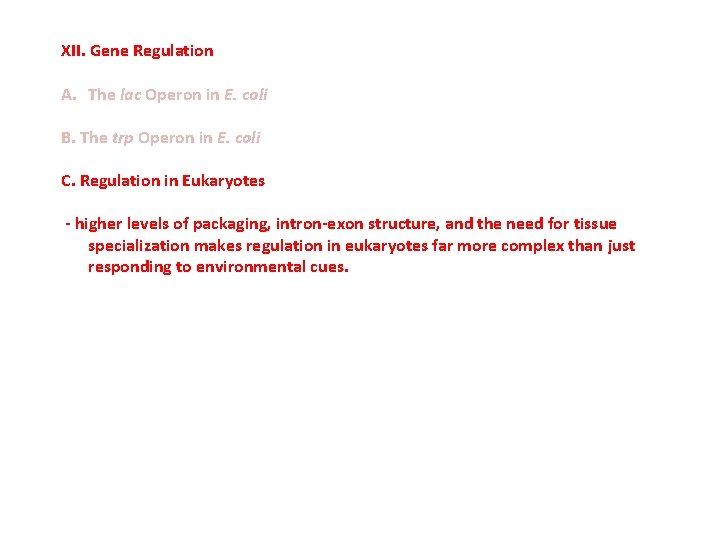
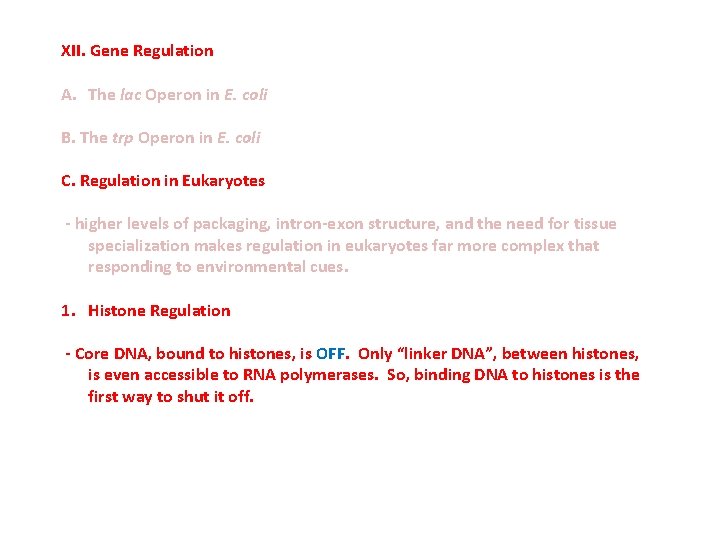
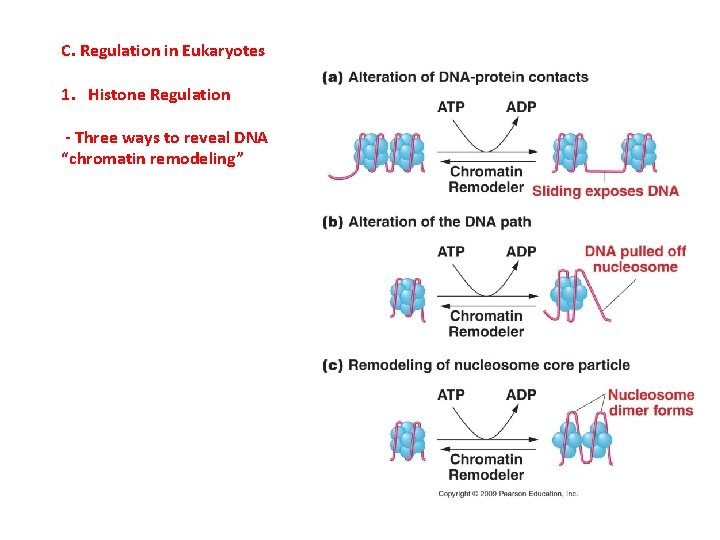
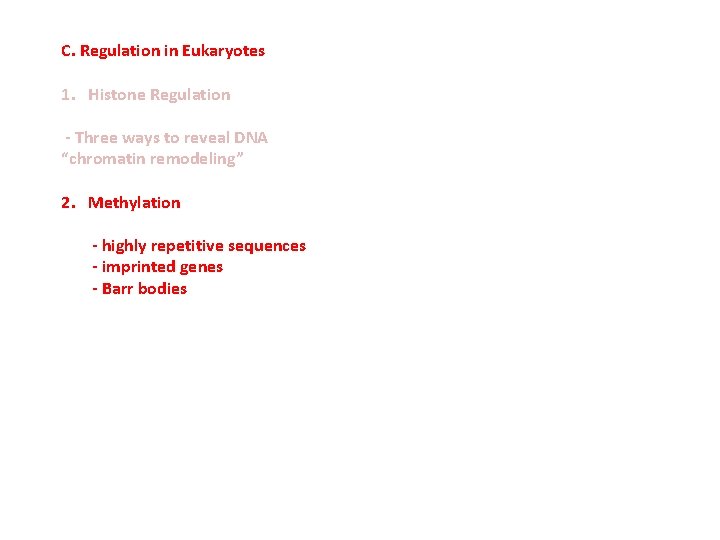
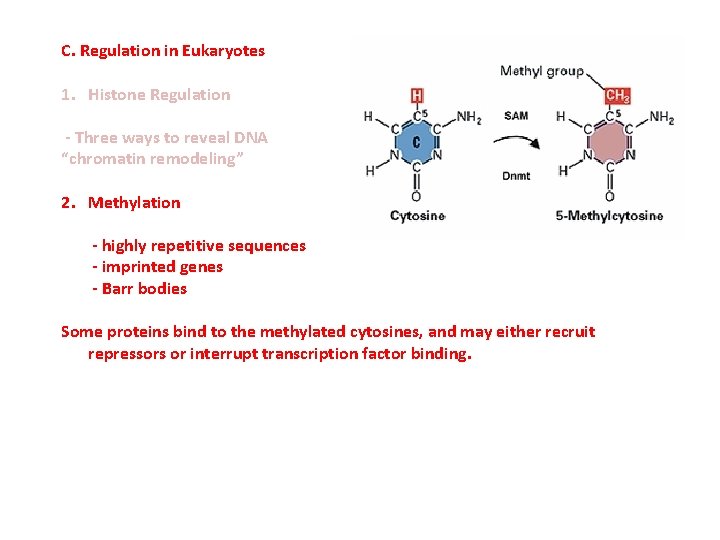
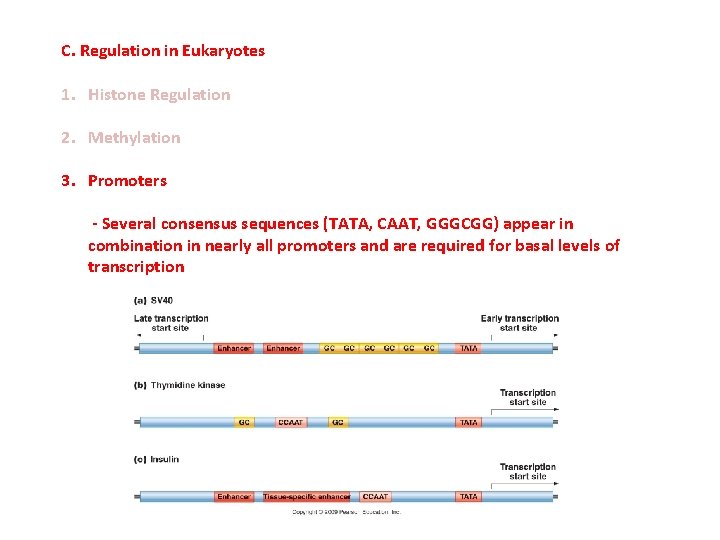
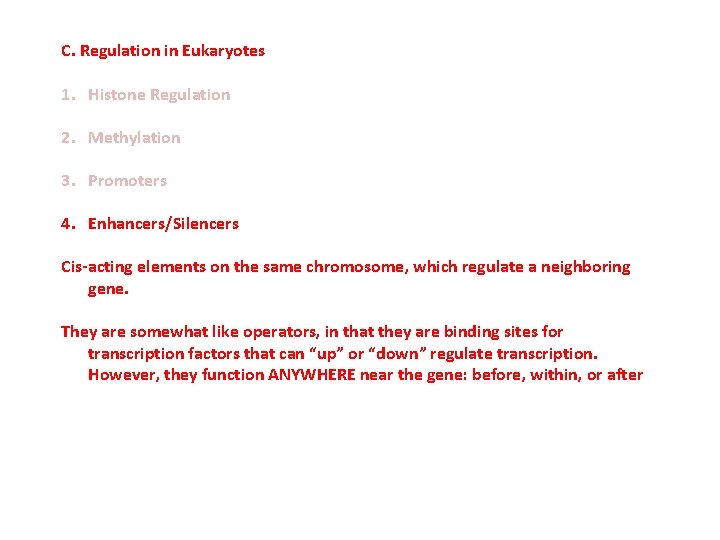
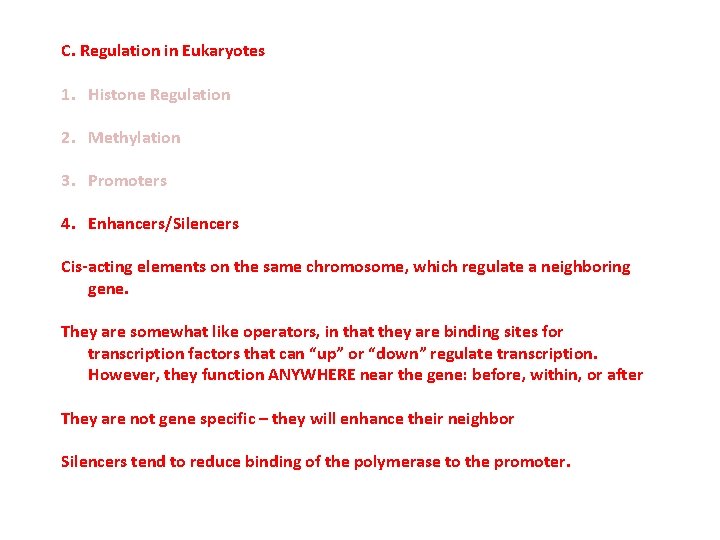
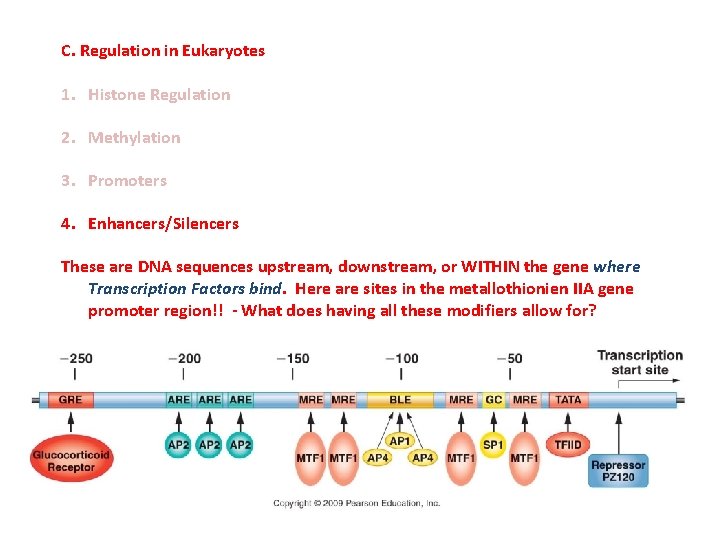
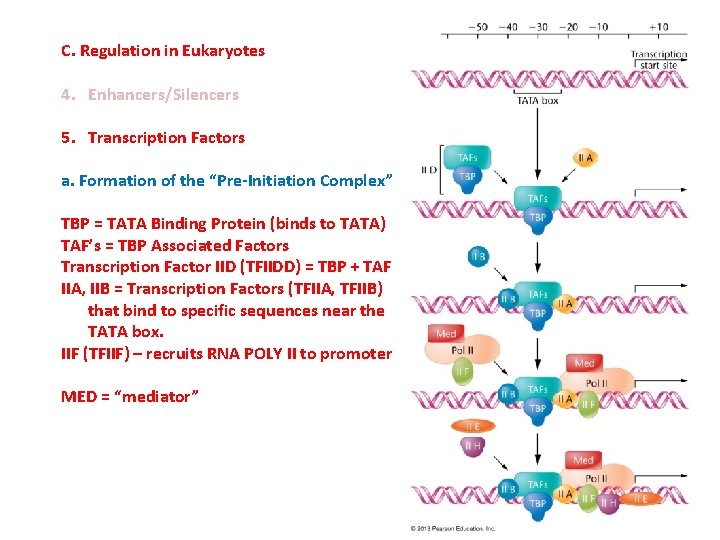
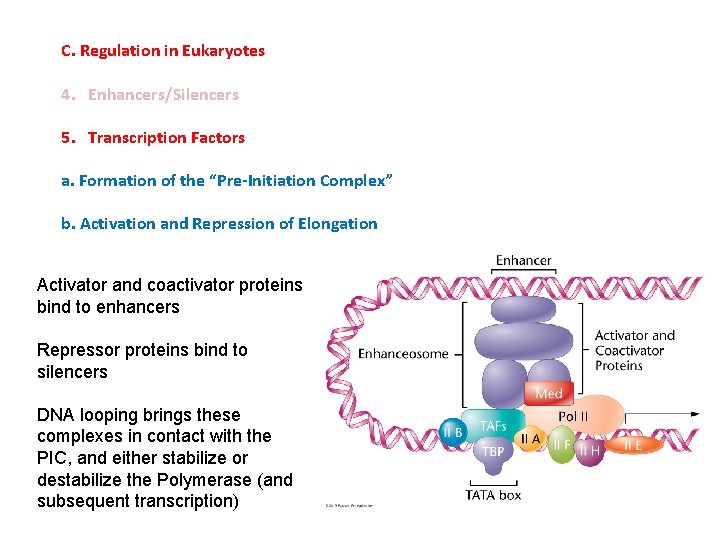
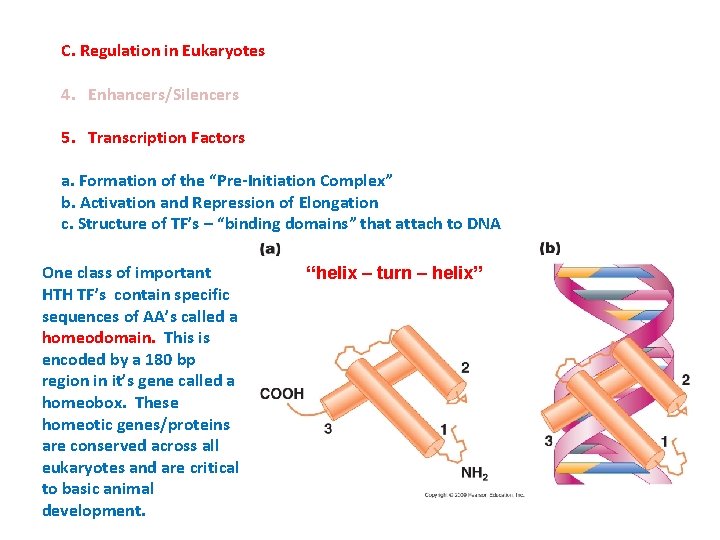
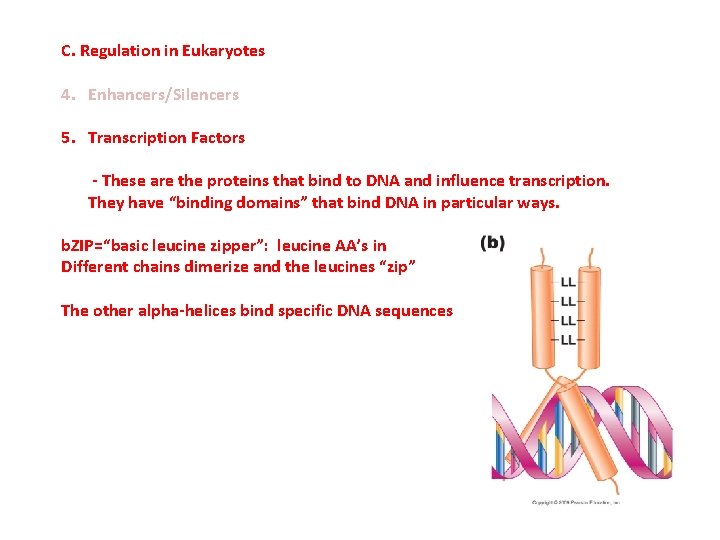
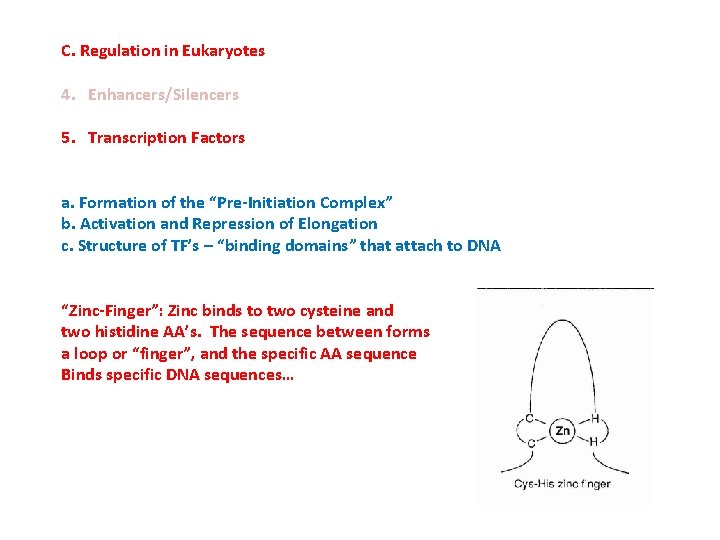
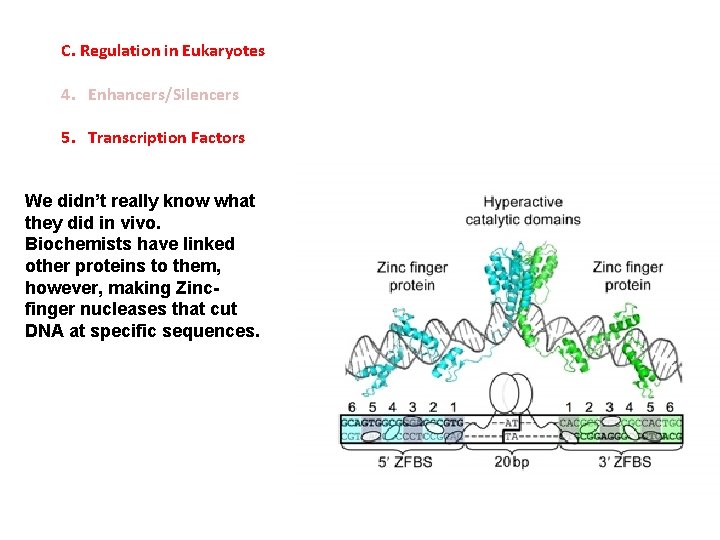
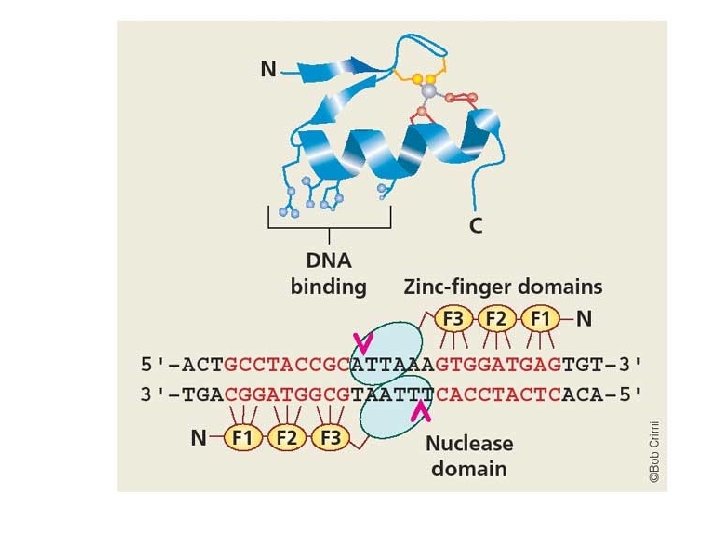
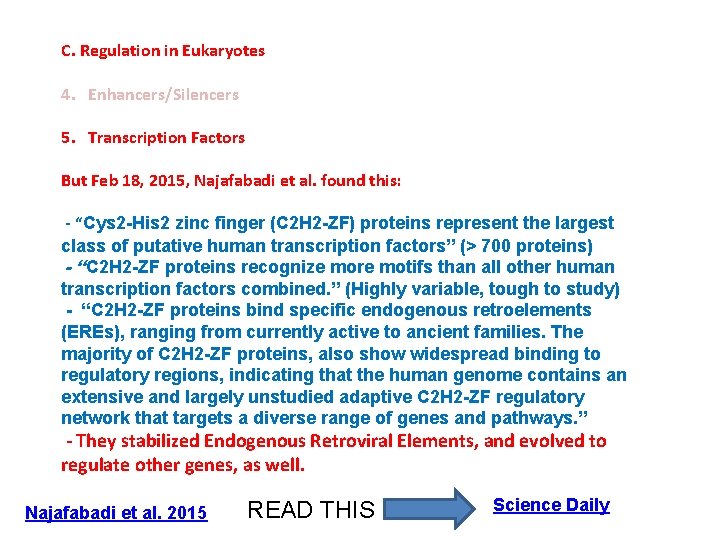
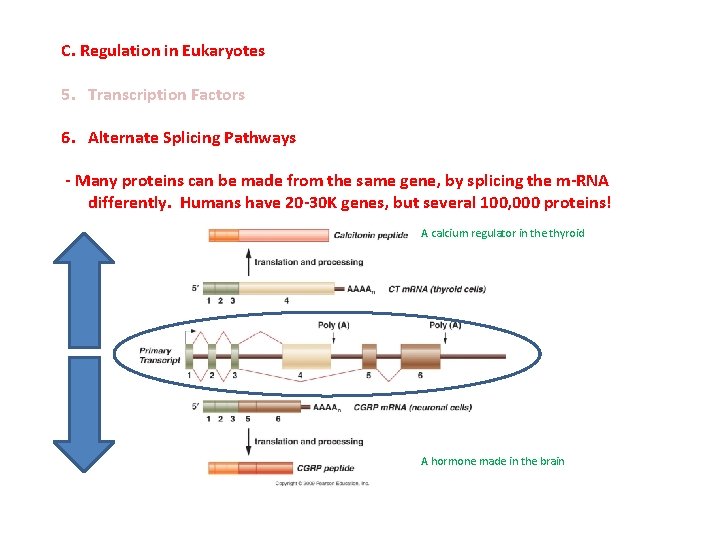
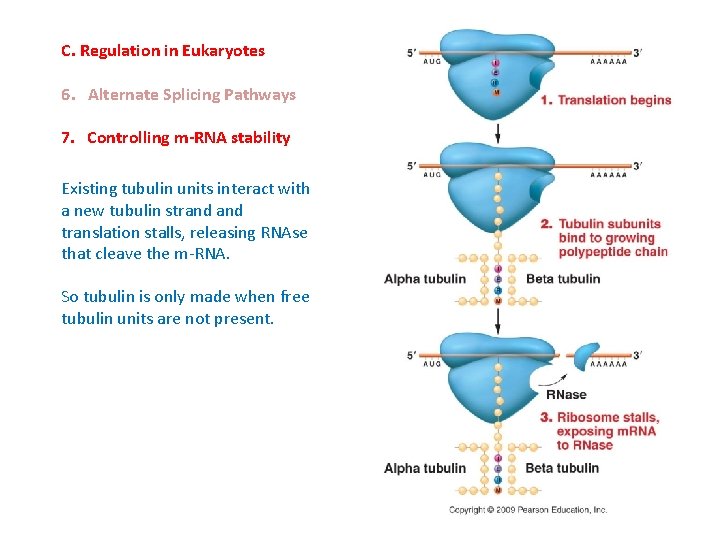
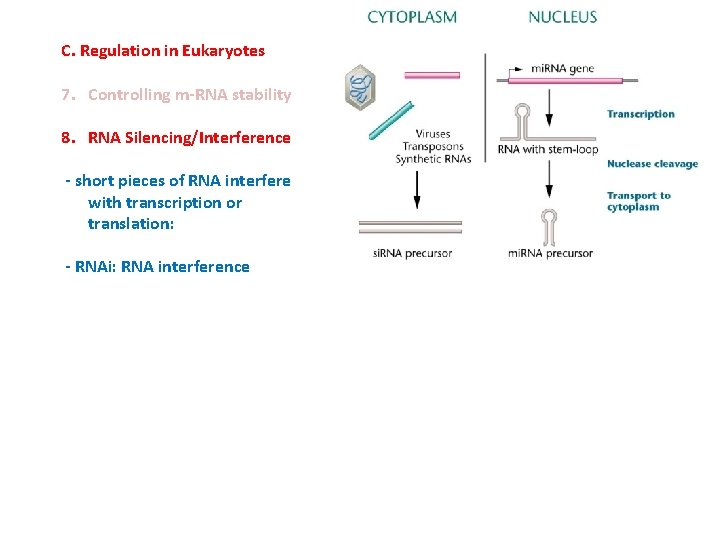
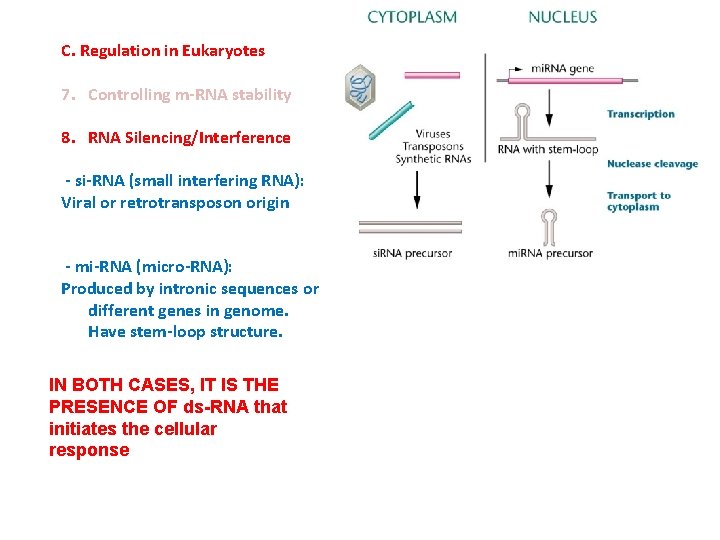
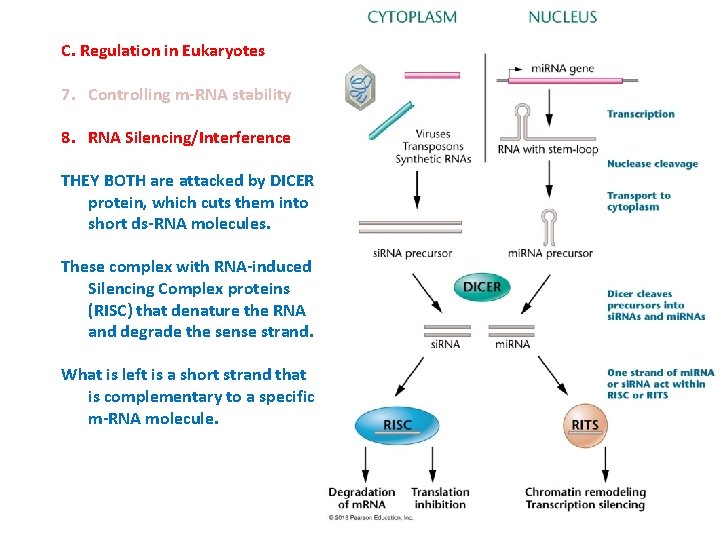
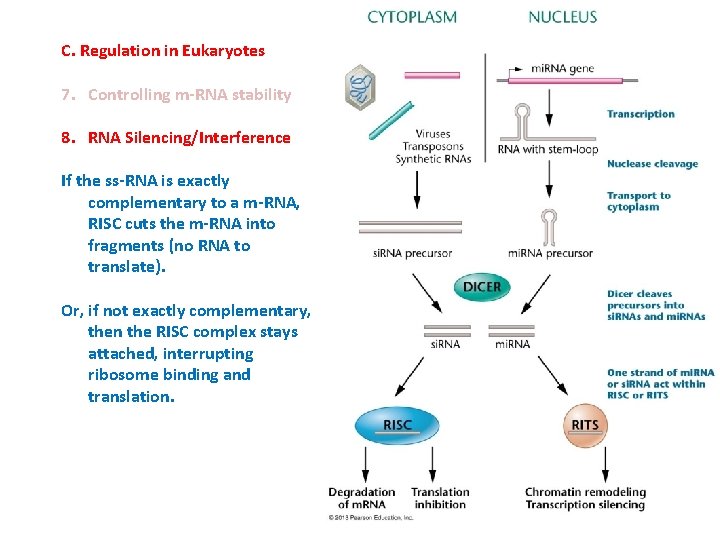
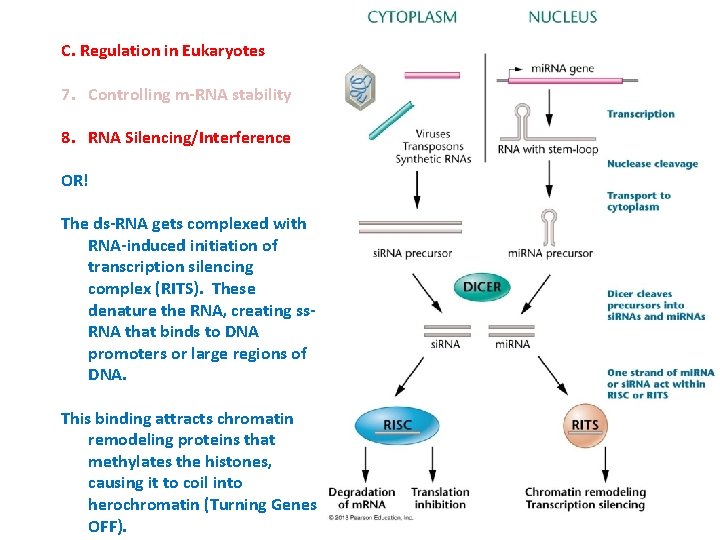
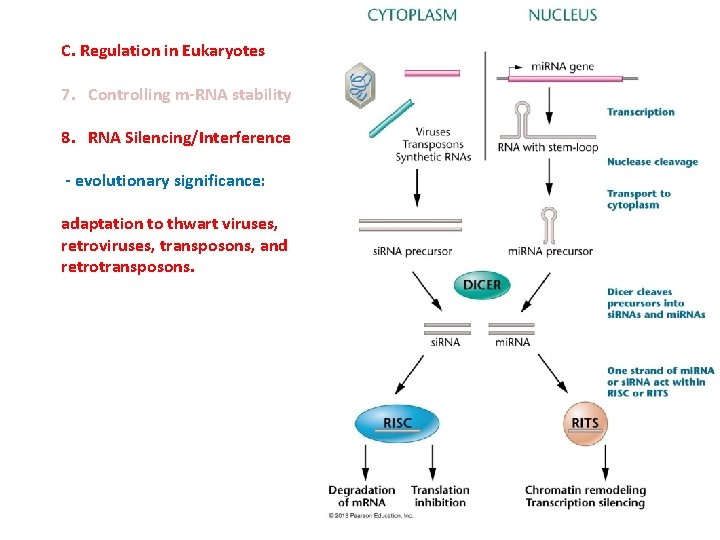
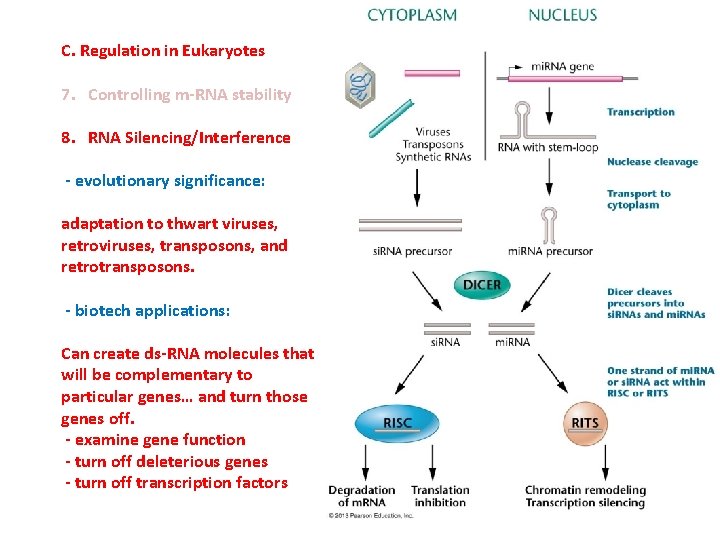
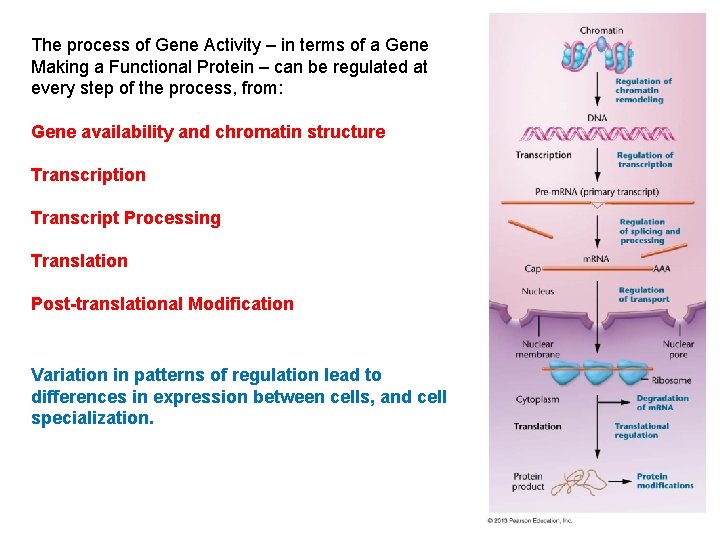
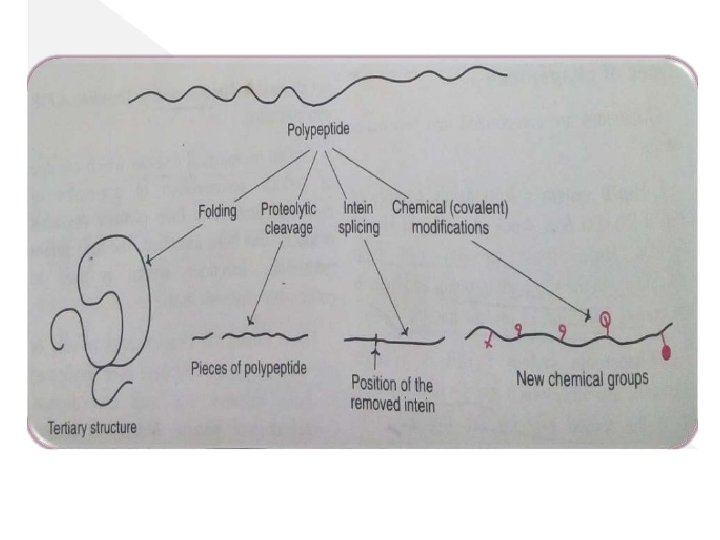
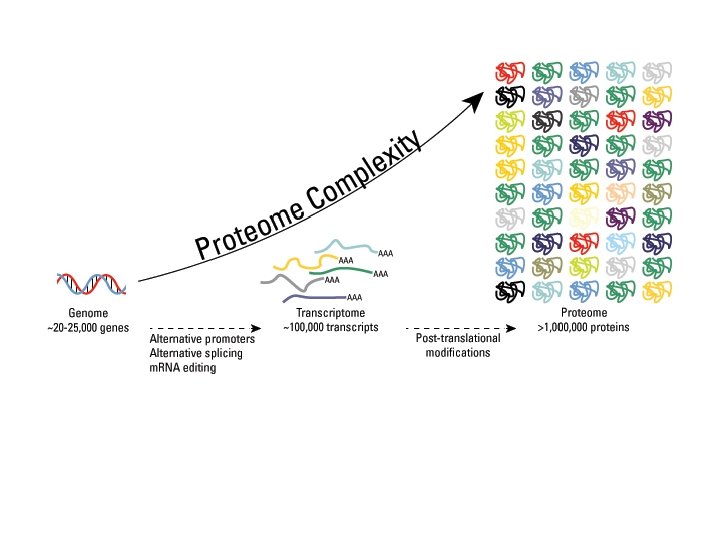
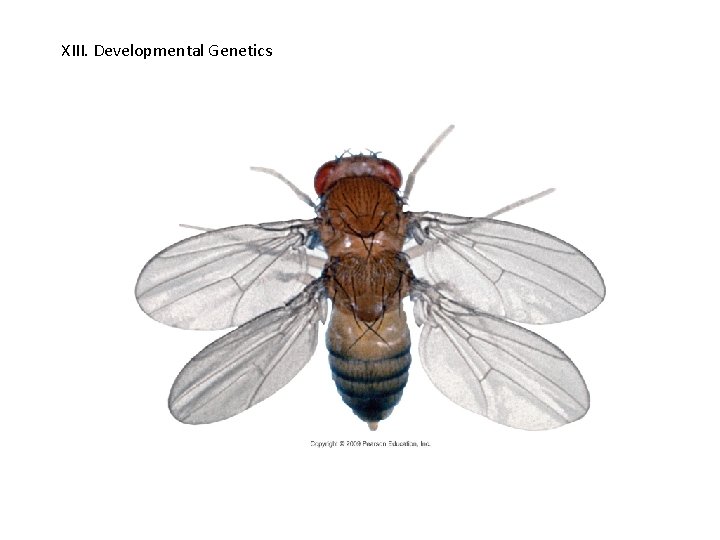
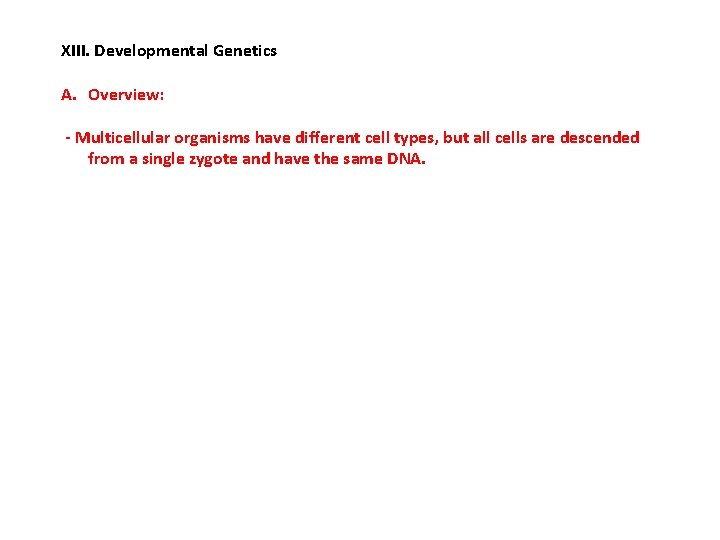
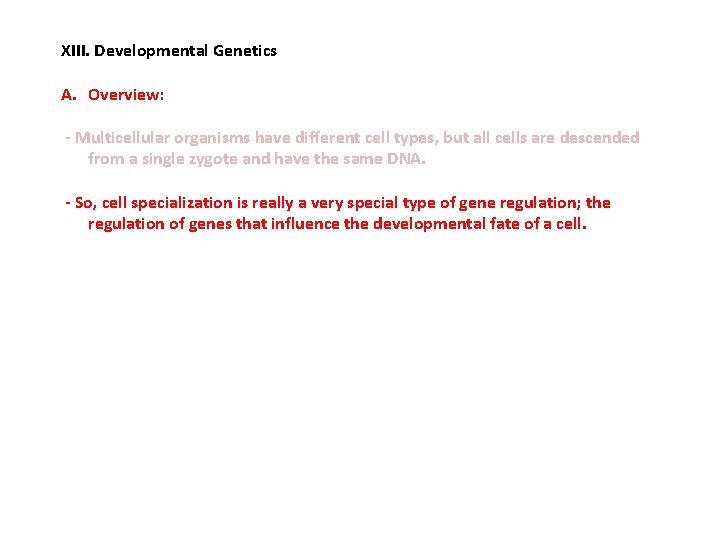
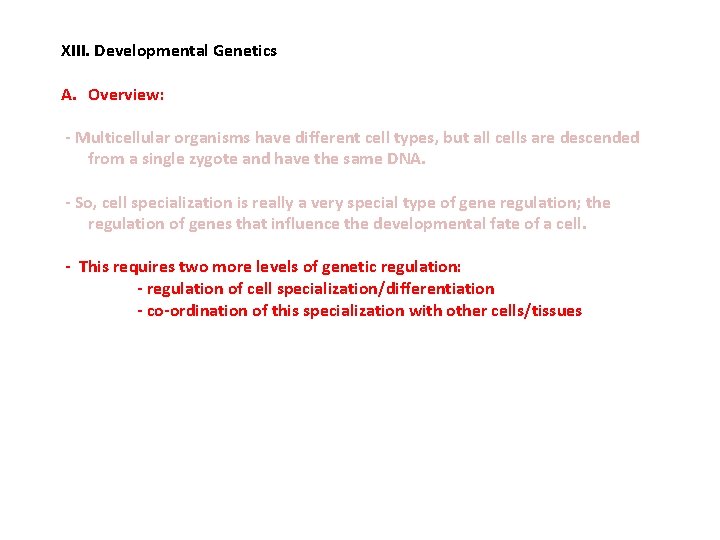
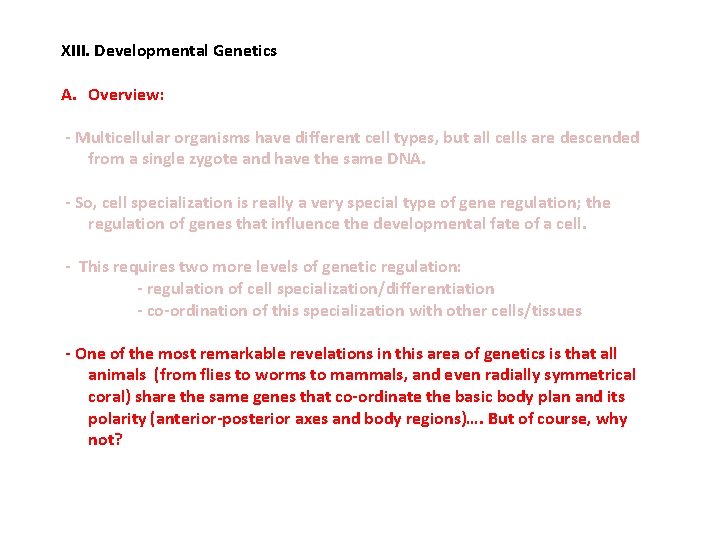
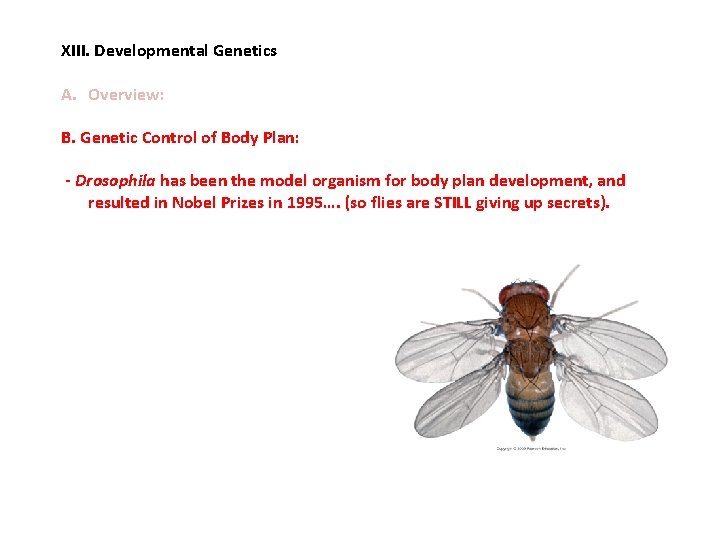
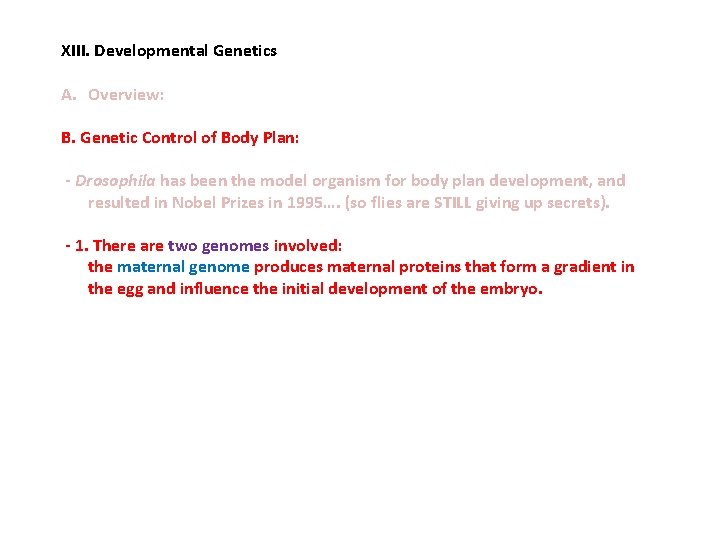
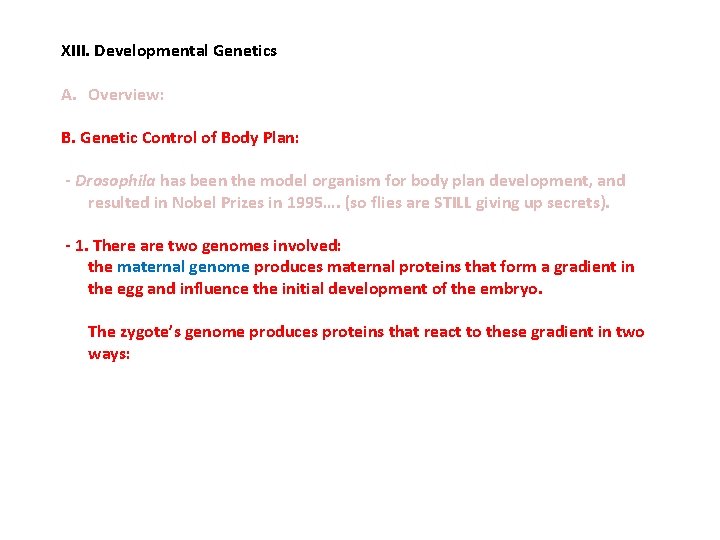
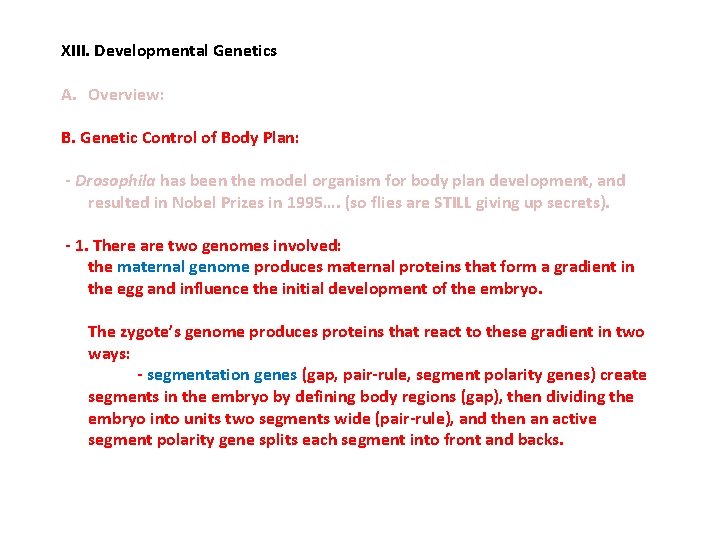
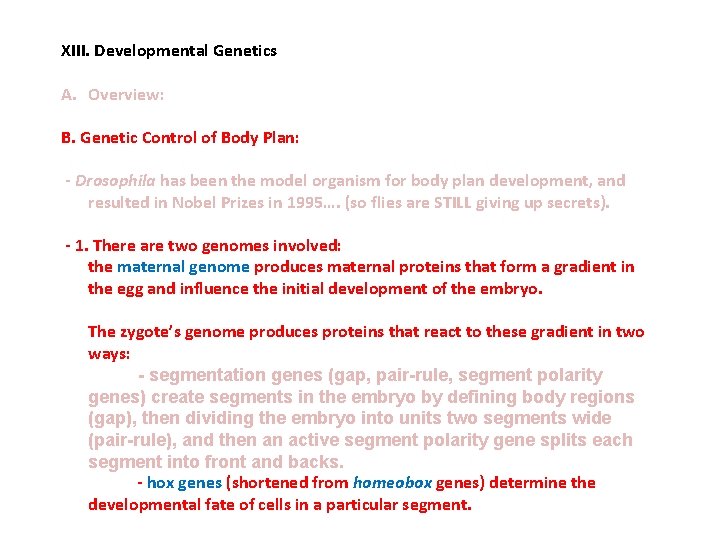
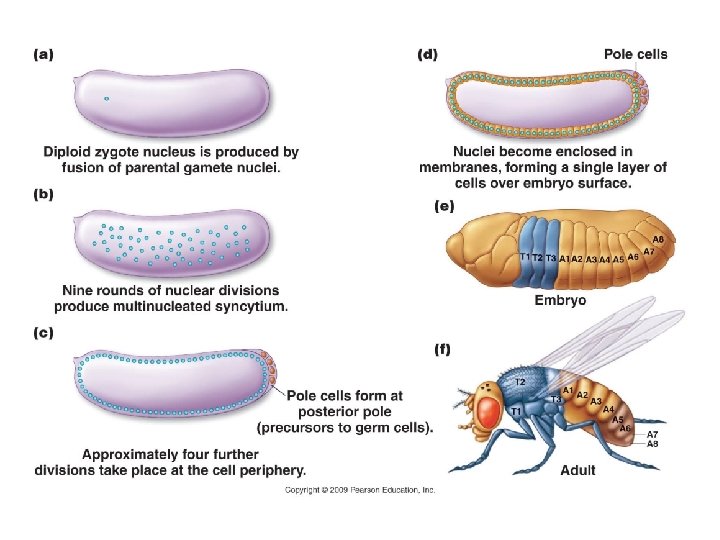
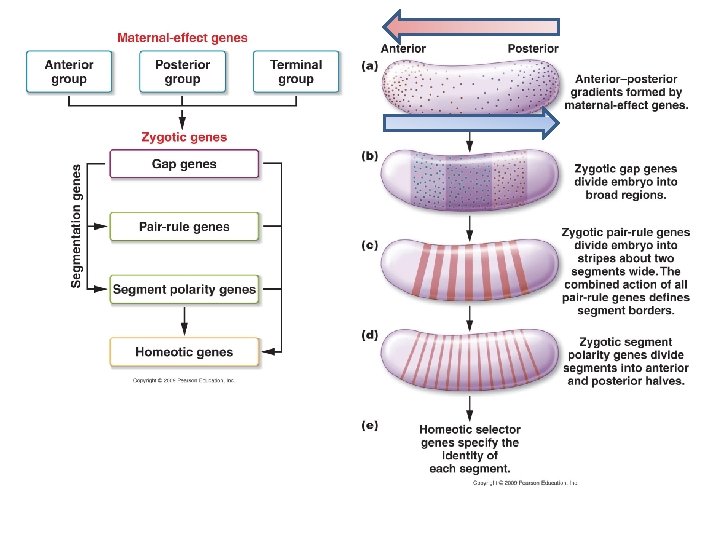
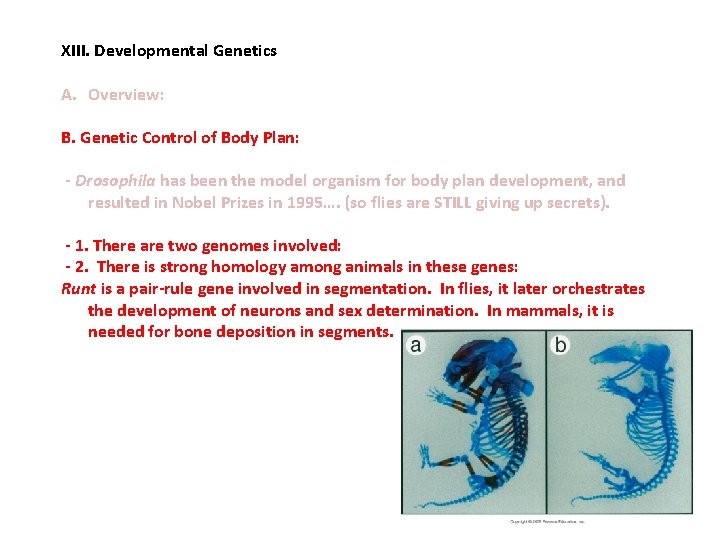
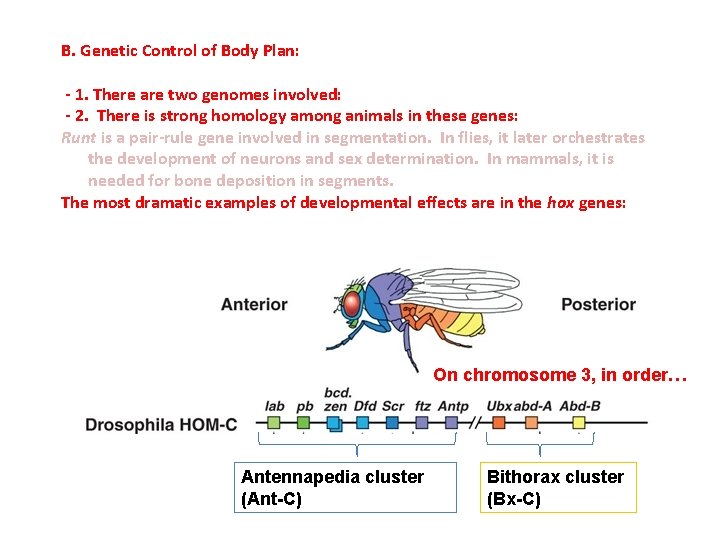
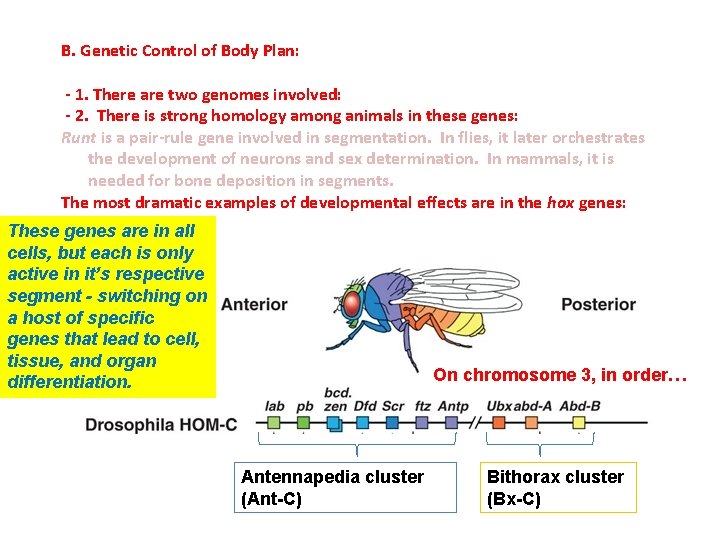
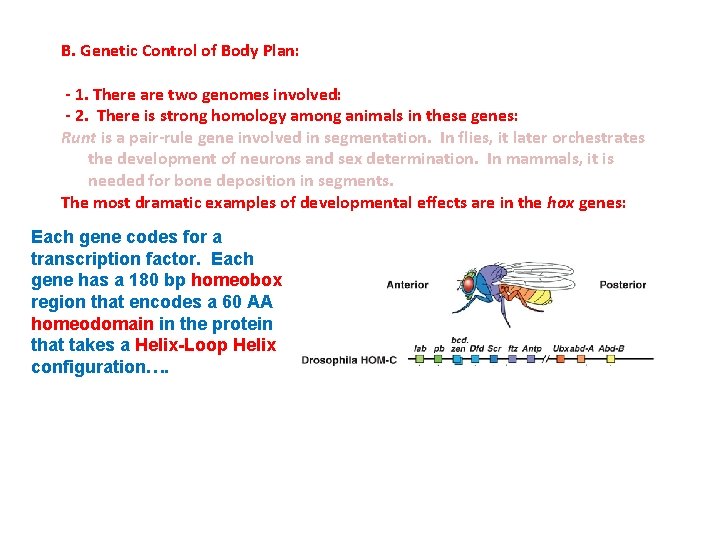
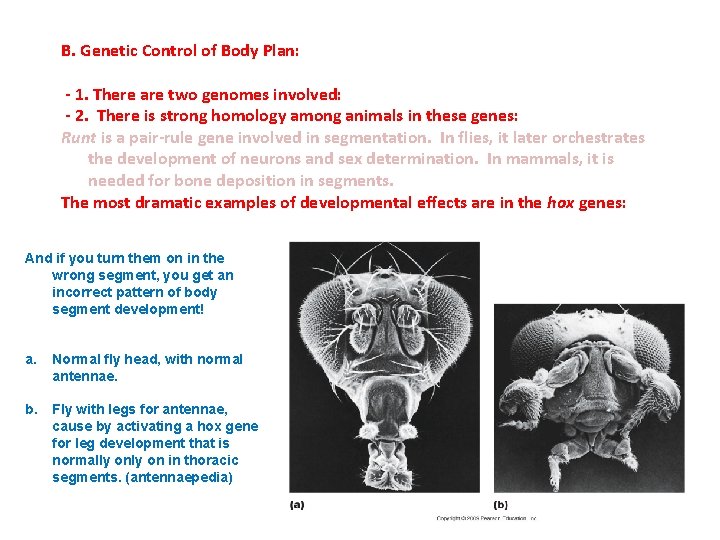
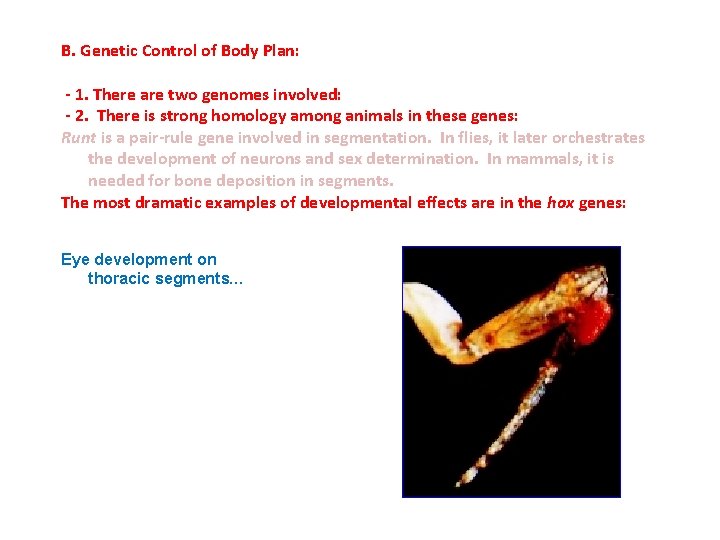
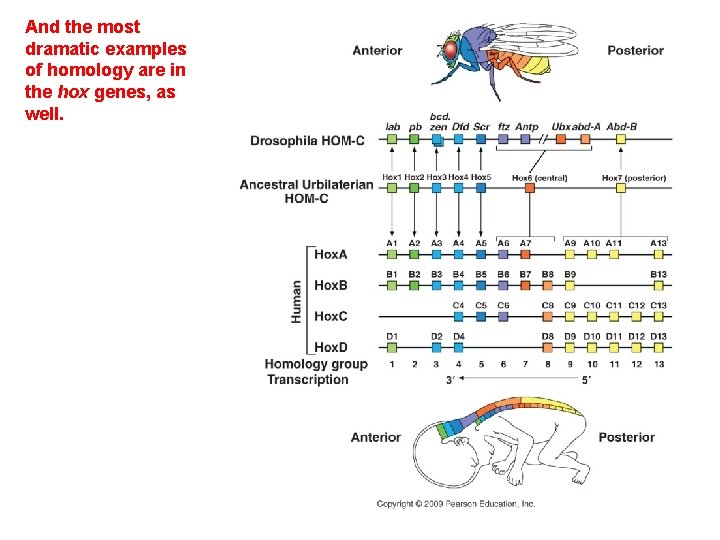
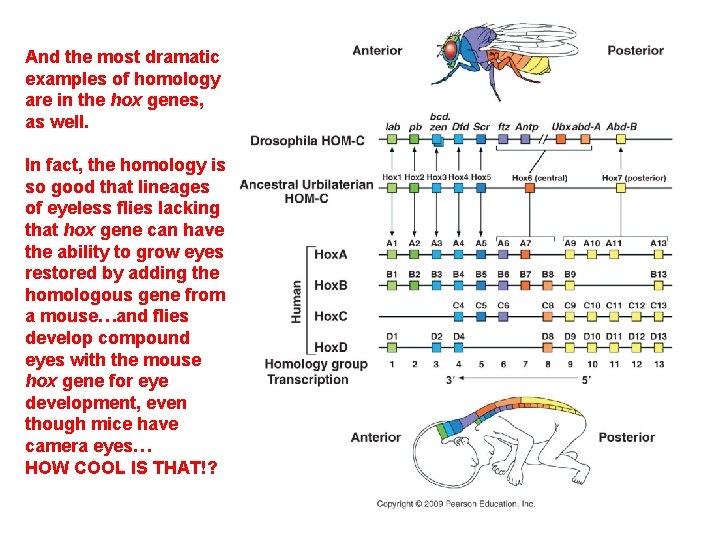
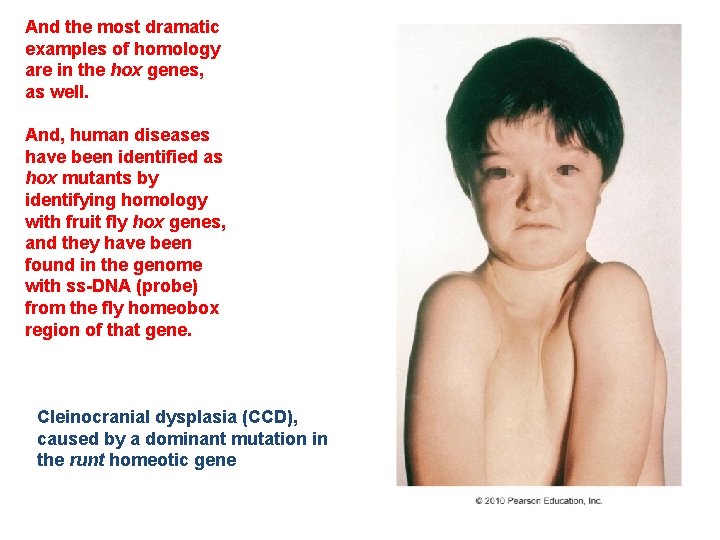
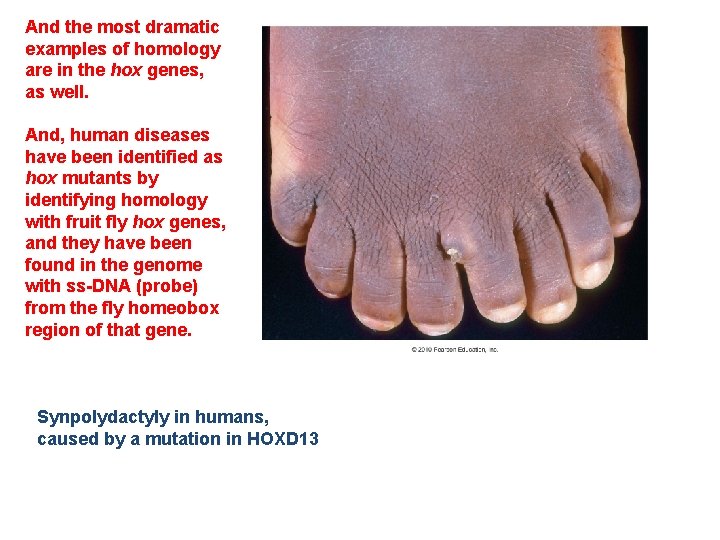
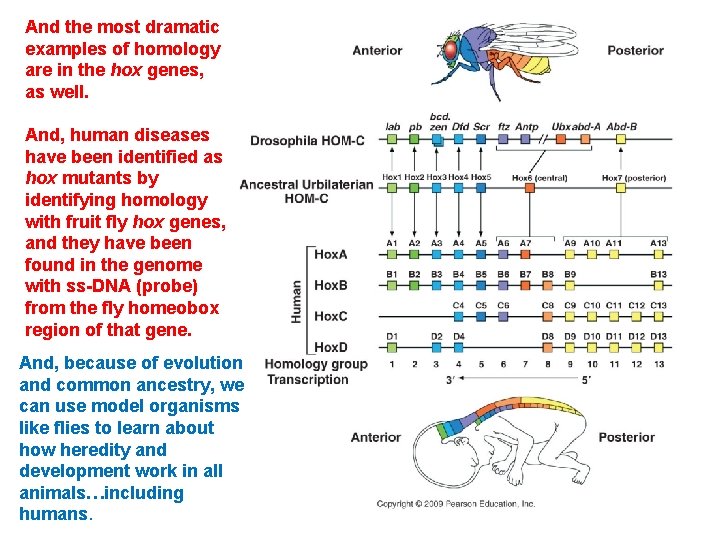
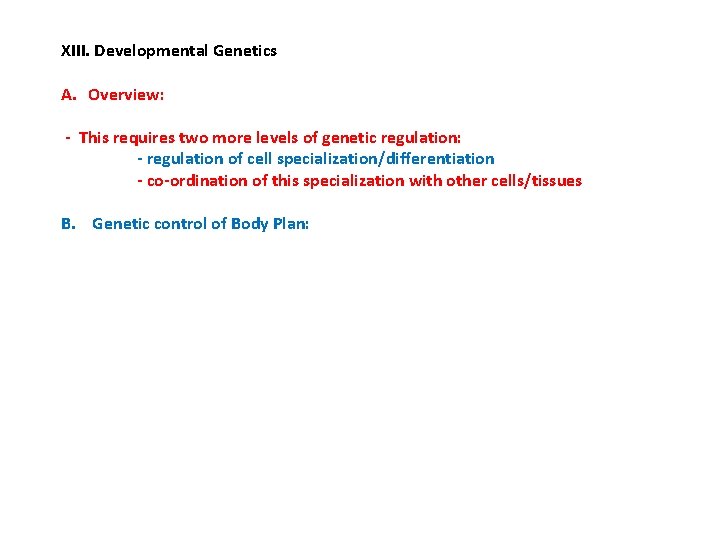
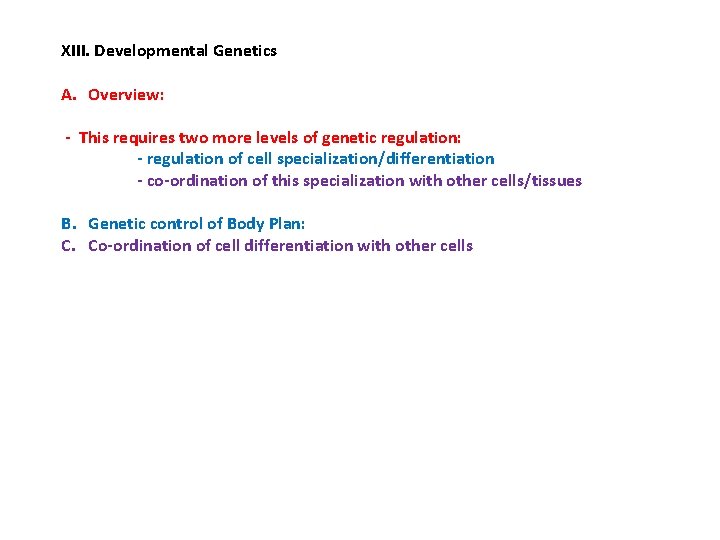
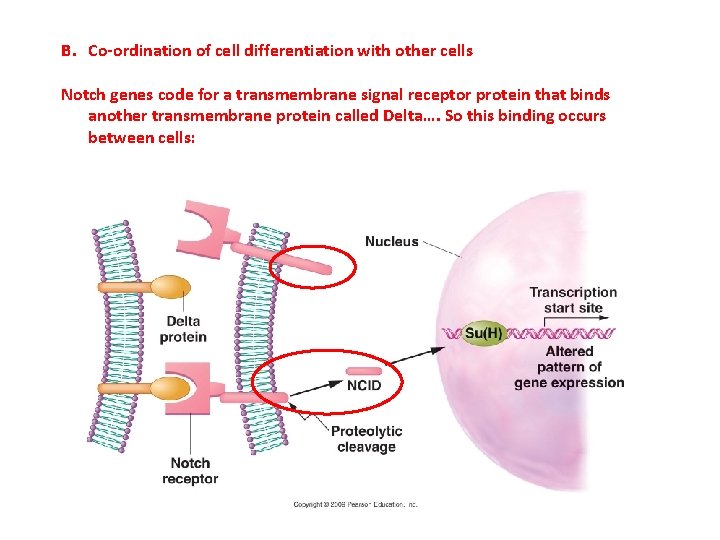
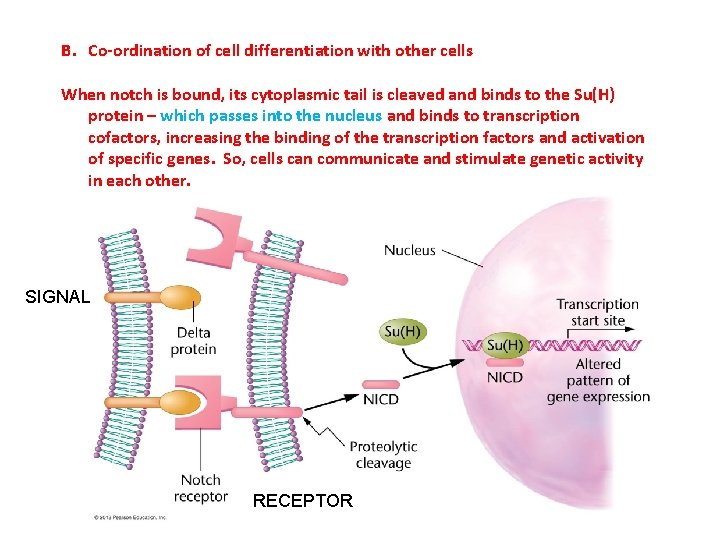
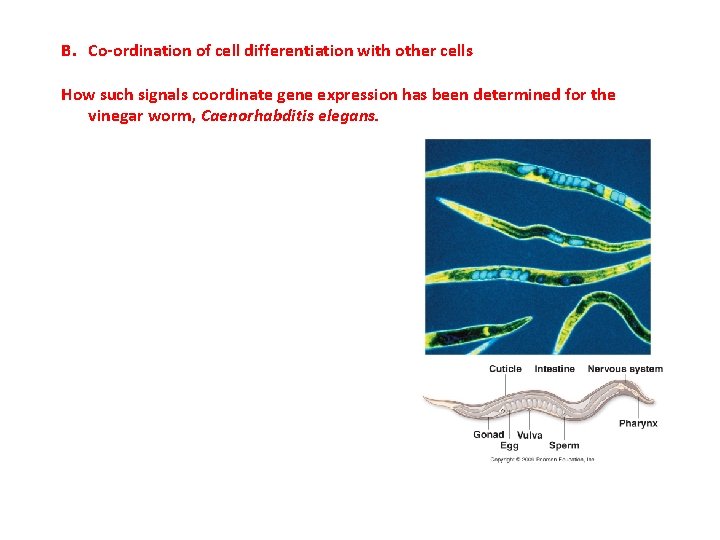
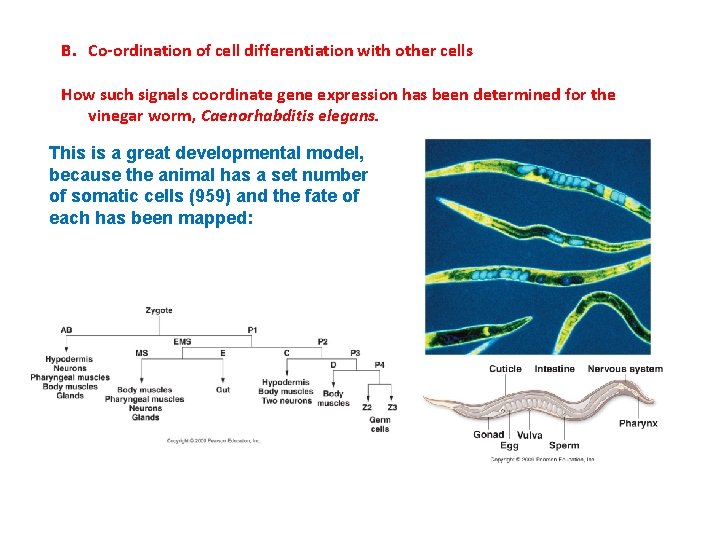
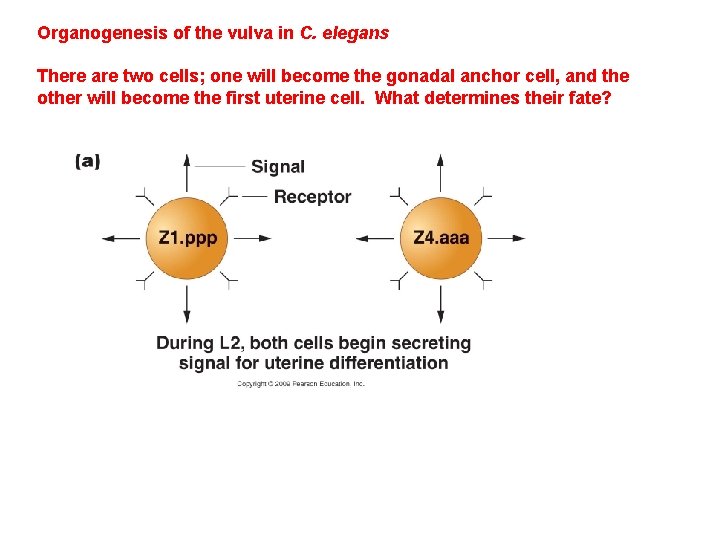
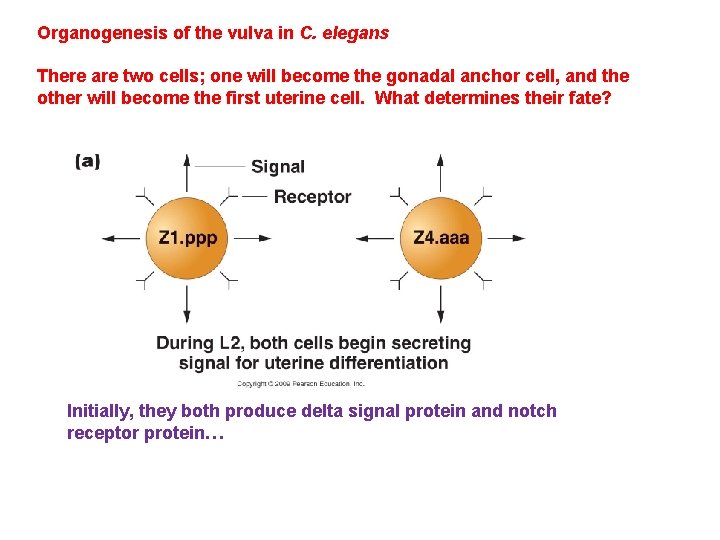
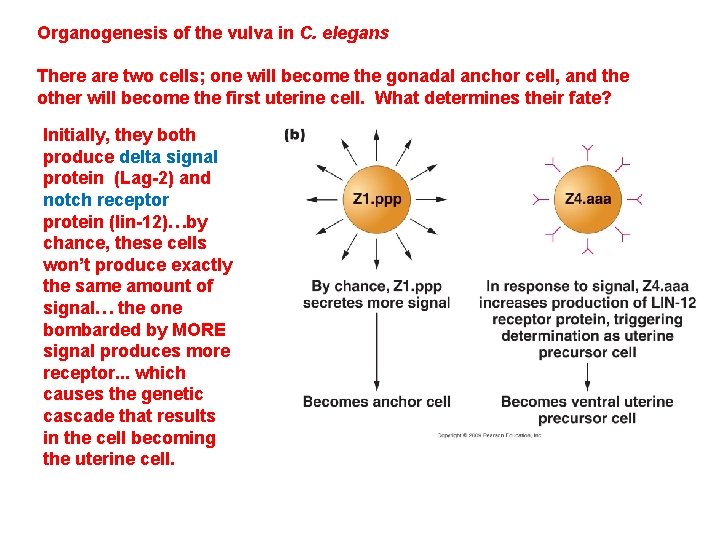
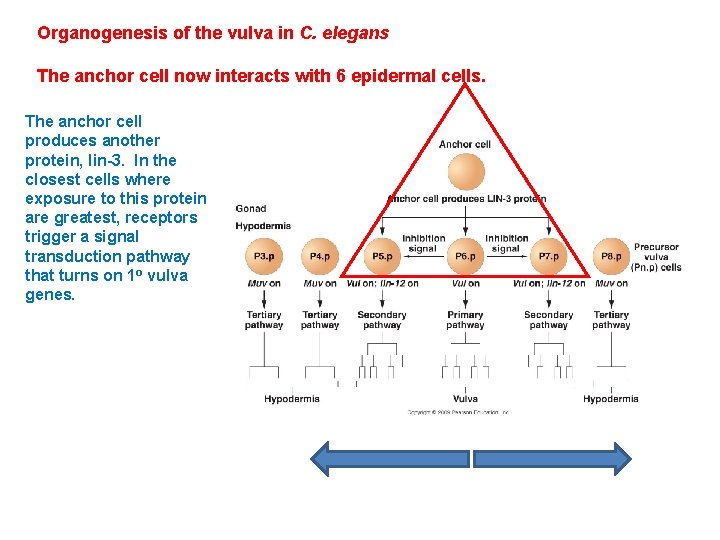
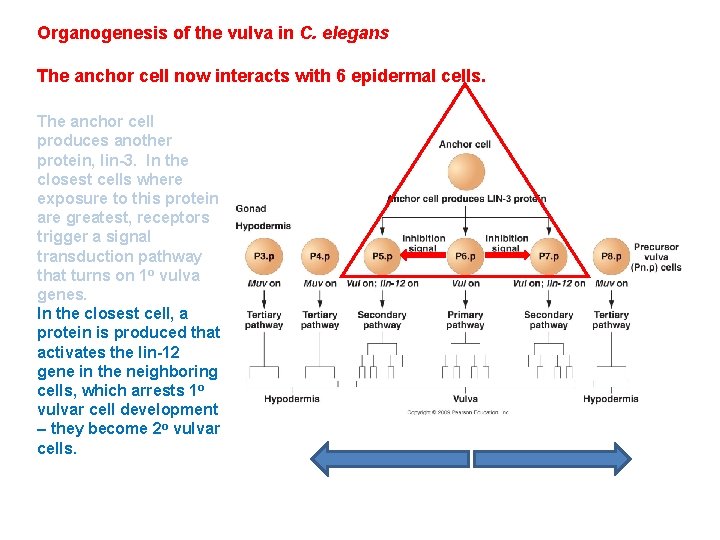
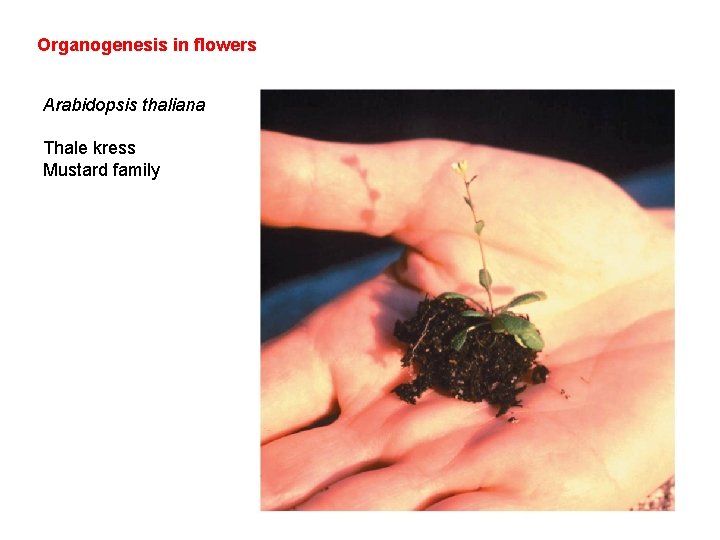
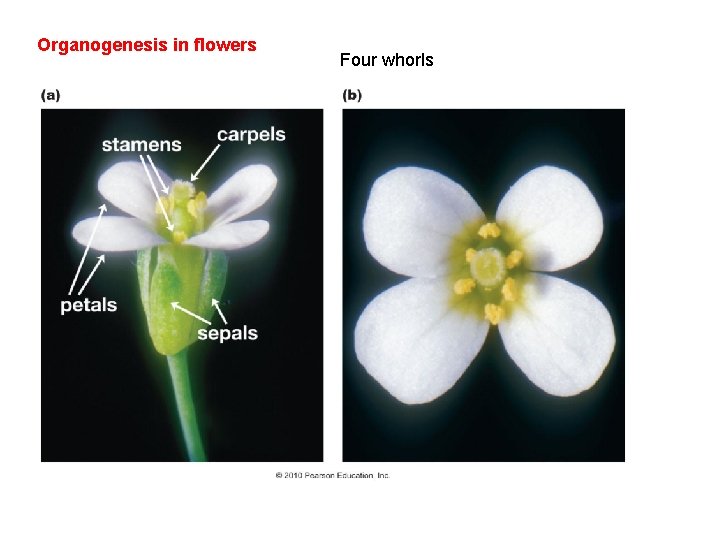
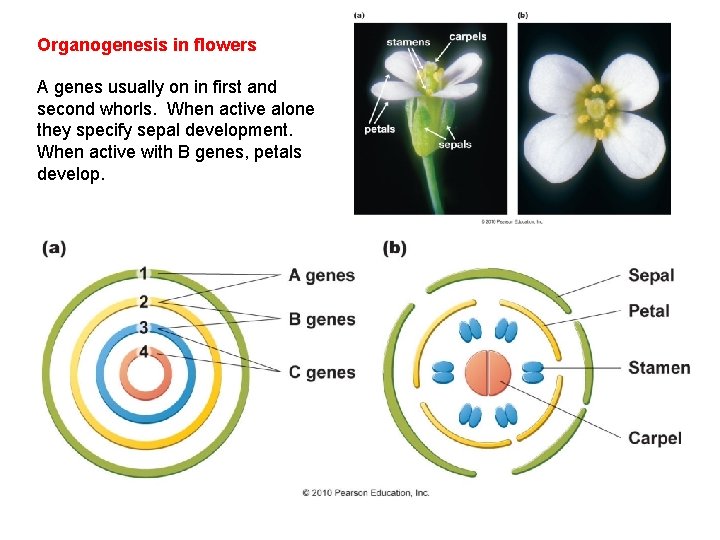
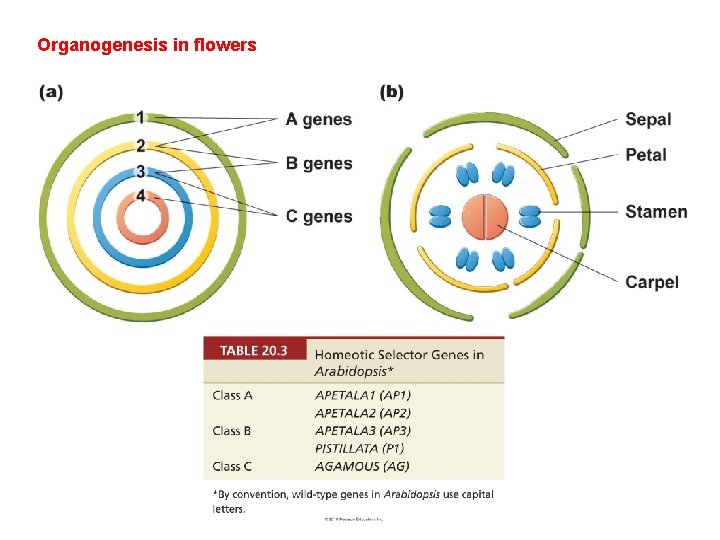
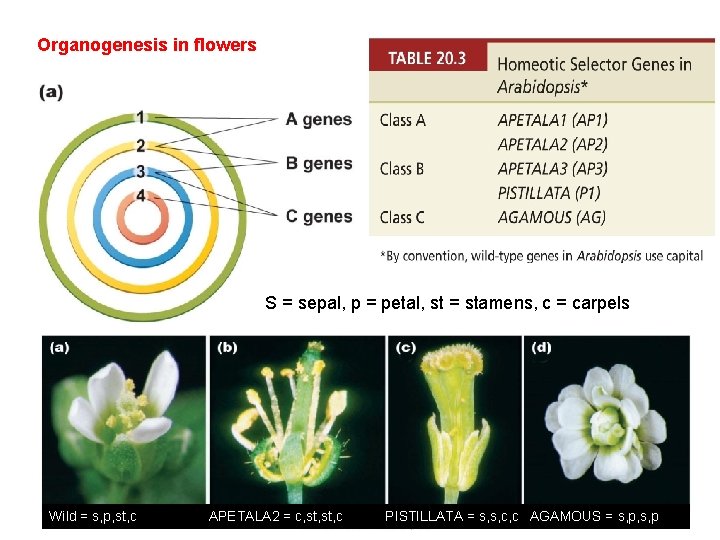
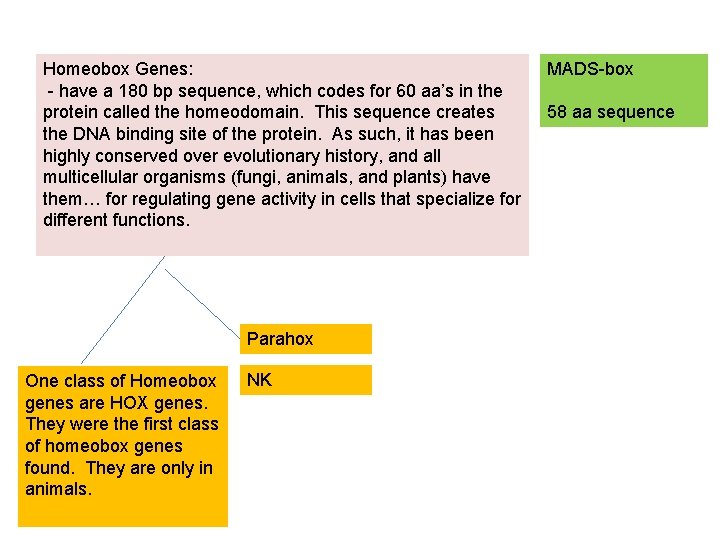
- Slides: 110
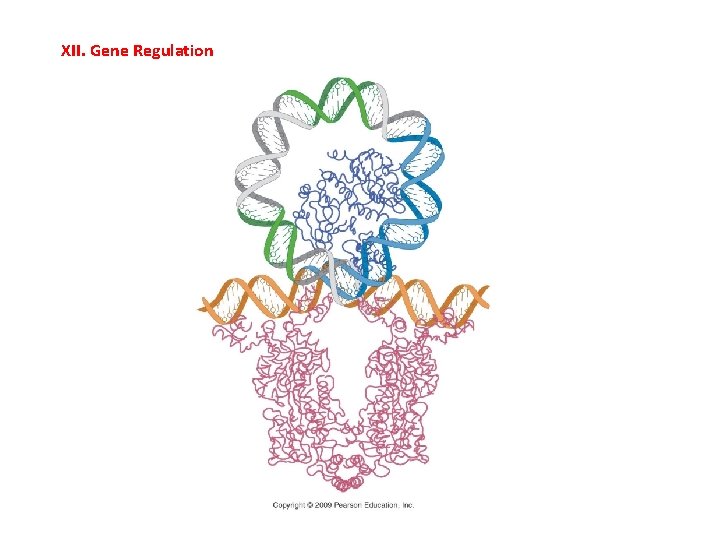
XII. Gene Regulation
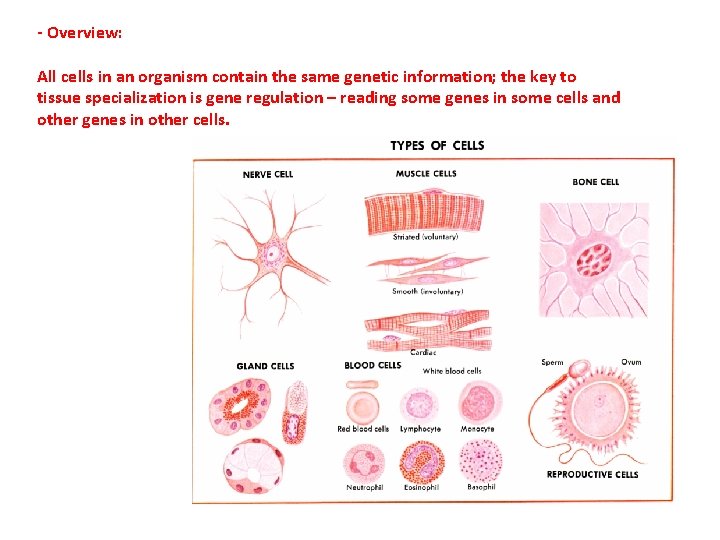
- Overview: All cells in an organism contain the same genetic information; the key to tissue specialization is gene regulation – reading some genes in some cells and other genes in other cells.
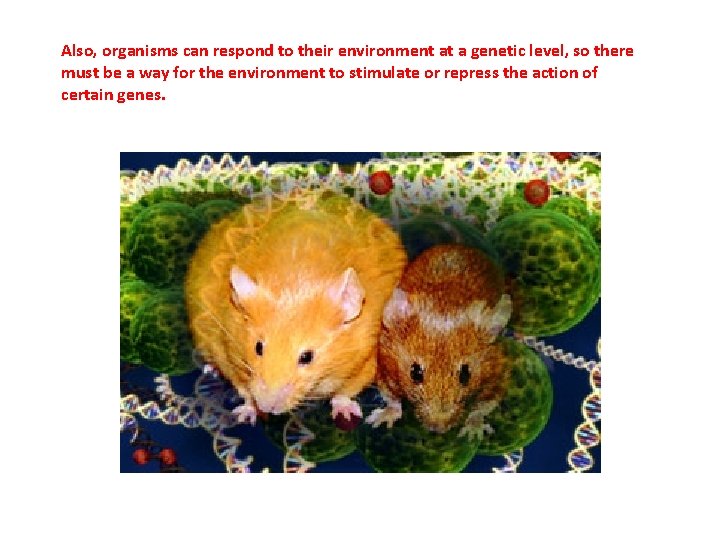
Also, organisms can respond to their environment at a genetic level, so there must be a way for the environment to stimulate or repress the action of certain genes.
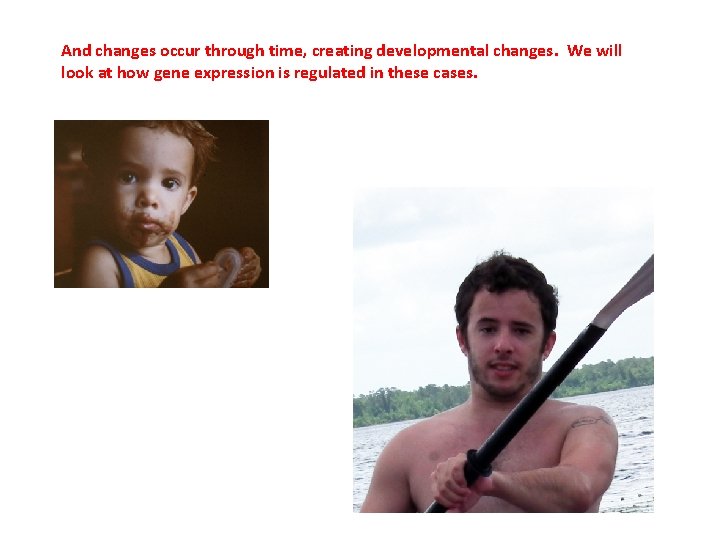
And changes occur through time, creating developmental changes. We will look at how gene expression is regulated in these cases.
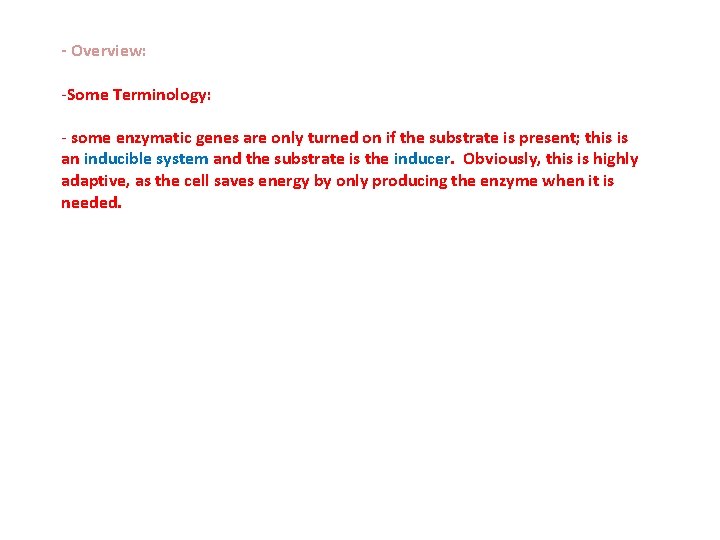
- Overview: -Some Terminology: - some enzymatic genes are only turned on if the substrate is present; this is an inducible system and the substrate is the inducer. Obviously, this is highly adaptive, as the cell saves energy by only producing the enzyme when it is needed.
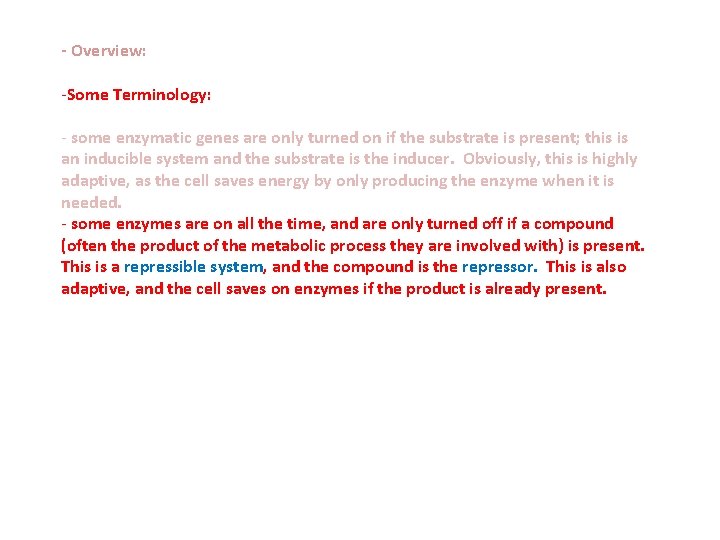
- Overview: -Some Terminology: - some enzymatic genes are only turned on if the substrate is present; this is an inducible system and the substrate is the inducer. Obviously, this is highly adaptive, as the cell saves energy by only producing the enzyme when it is needed. - some enzymes are on all the time, and are only turned off if a compound (often the product of the metabolic process they are involved with) is present. This is a repressible system, and the compound is the repressor. This is also adaptive, and the cell saves on enzymes if the product is already present.
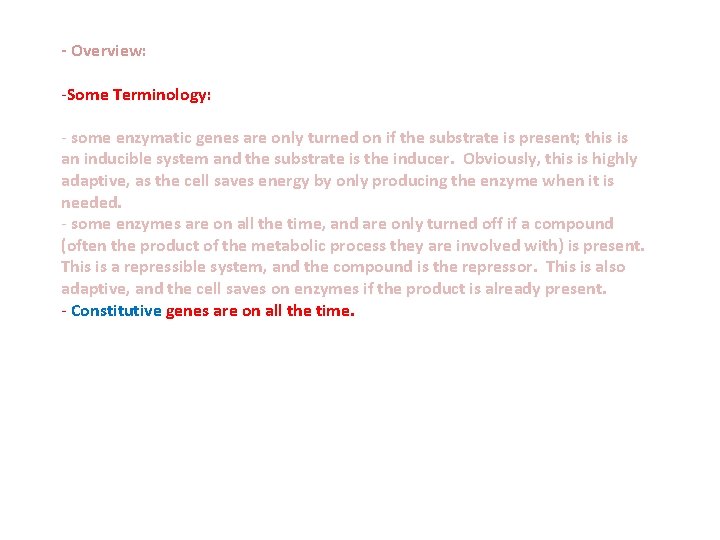
- Overview: -Some Terminology: - some enzymatic genes are only turned on if the substrate is present; this is an inducible system and the substrate is the inducer. Obviously, this is highly adaptive, as the cell saves energy by only producing the enzyme when it is needed. - some enzymes are on all the time, and are only turned off if a compound (often the product of the metabolic process they are involved with) is present. This is a repressible system, and the compound is the repressor. This is also adaptive, and the cell saves on enzymes if the product is already present. - Constitutive genes are on all the time.
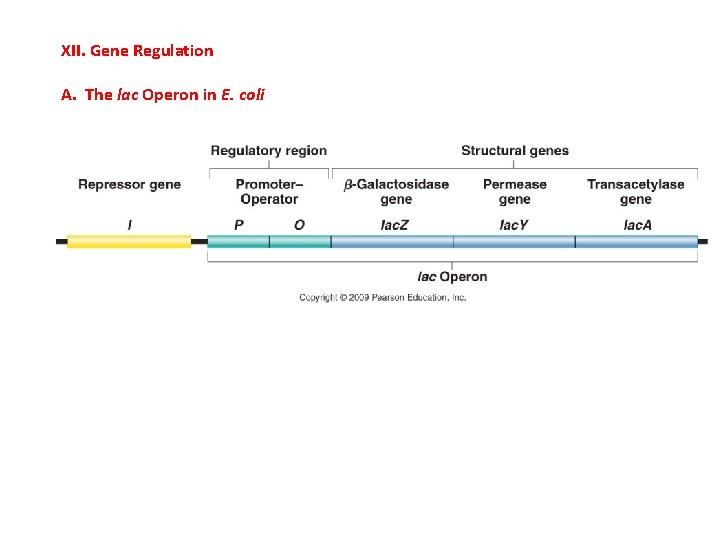
XII. Gene Regulation A. The lac Operon in E. coli
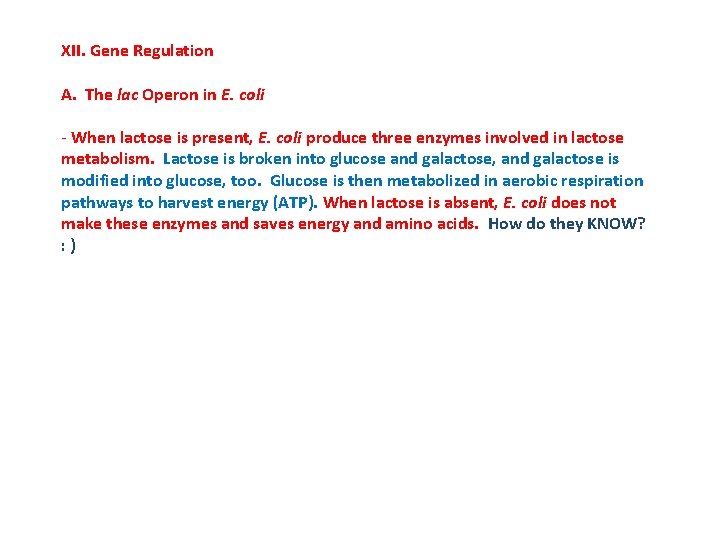
XII. Gene Regulation A. The lac Operon in E. coli - When lactose is present, E. coli produce three enzymes involved in lactose metabolism. Lactose is broken into glucose and galactose, and galactose is modified into glucose, too. Glucose is then metabolized in aerobic respiration pathways to harvest energy (ATP). When lactose is absent, E. coli does not make these enzymes and saves energy and amino acids. How do they KNOW? : )
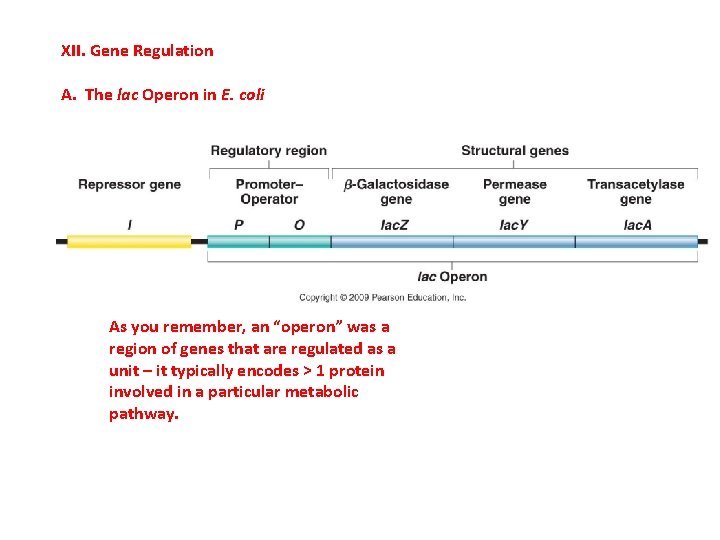
XII. Gene Regulation A. The lac Operon in E. coli As you remember, an “operon” was a region of genes that are regulated as a unit – it typically encodes > 1 protein involved in a particular metabolic pathway.
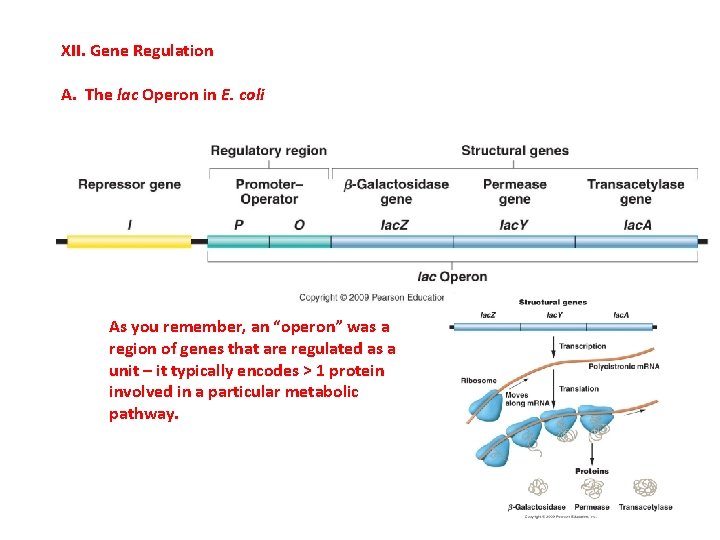
XII. Gene Regulation A. The lac Operon in E. coli As you remember, an “operon” was a region of genes that are regulated as a unit – it typically encodes > 1 protein involved in a particular metabolic pathway.
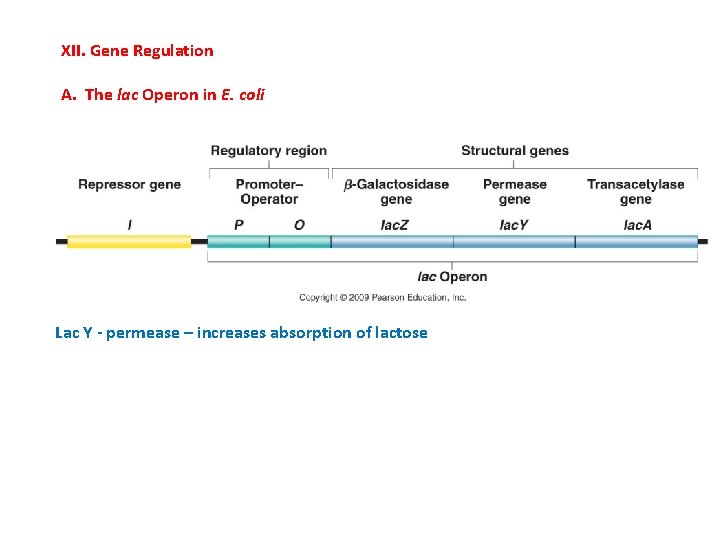
XII. Gene Regulation A. The lac Operon in E. coli Lac Y - permease – increases absorption of lactose
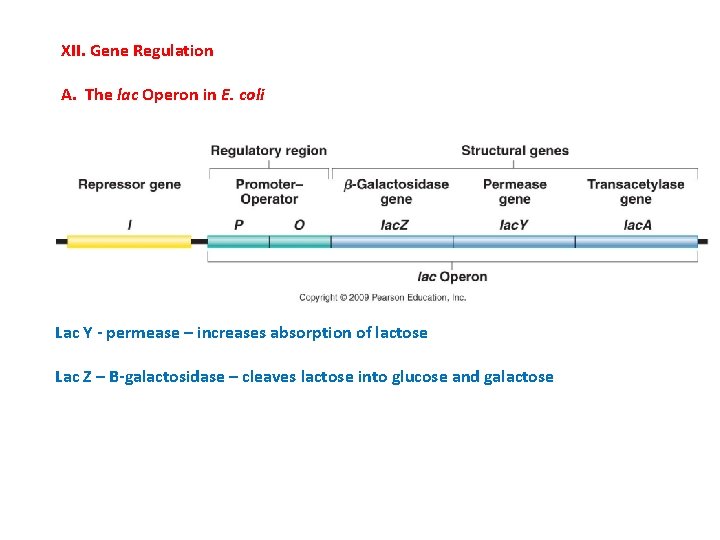
XII. Gene Regulation A. The lac Operon in E. coli Lac Y - permease – increases absorption of lactose Lac Z – B-galactosidase – cleaves lactose into glucose and galactose
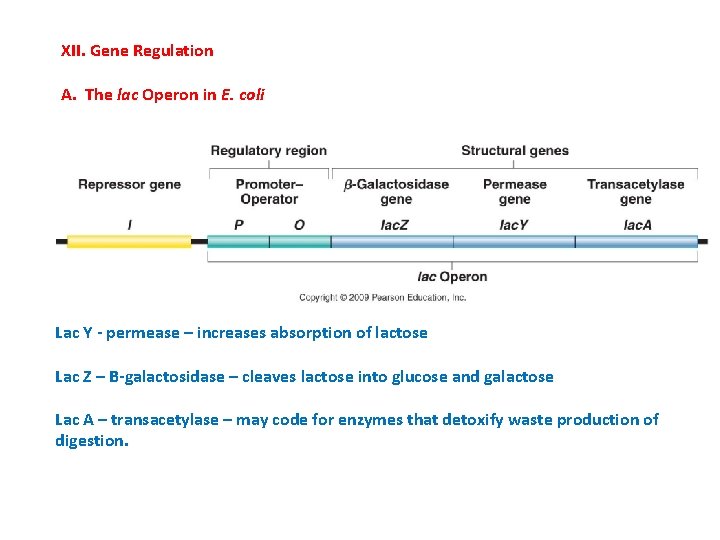
XII. Gene Regulation A. The lac Operon in E. coli Lac Y - permease – increases absorption of lactose Lac Z – B-galactosidase – cleaves lactose into glucose and galactose Lac A – transacetylase – may code for enzymes that detoxify waste production of digestion.
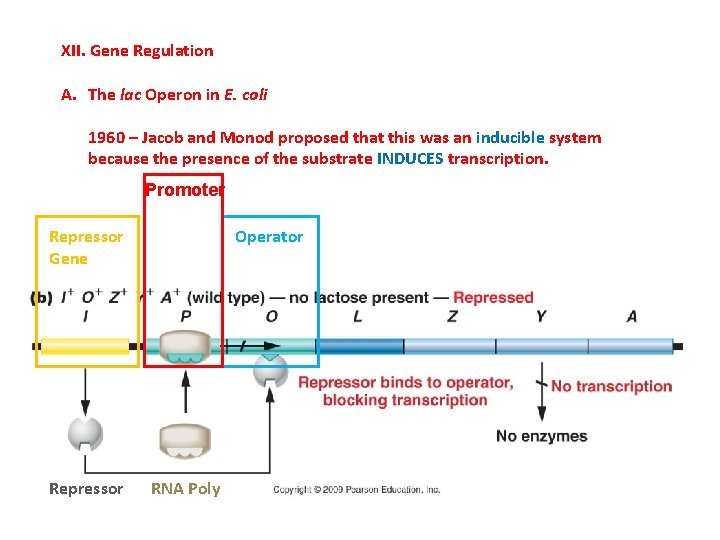
XII. Gene Regulation A. The lac Operon in E. coli 1960 – Jacob and Monod proposed that this was an inducible system because the presence of the substrate INDUCES transcription. Promoter Repressor Gene Repressor Operator RNA Poly
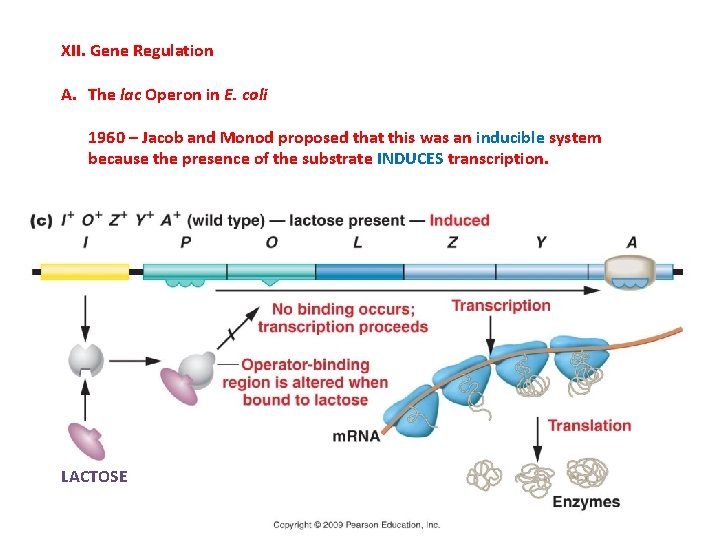
XII. Gene Regulation A. The lac Operon in E. coli 1960 – Jacob and Monod proposed that this was an inducible system because the presence of the substrate INDUCES transcription. LACTOSE
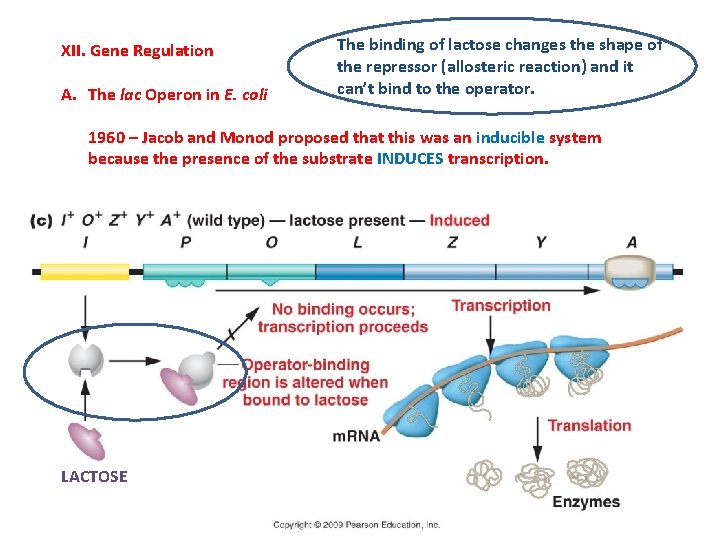
XII. Gene Regulation A. The lac Operon in E. coli The binding of lactose changes the shape of the repressor (allosteric reaction) and it can’t bind to the operator. 1960 – Jacob and Monod proposed that this was an inducible system because the presence of the substrate INDUCES transcription. LACTOSE
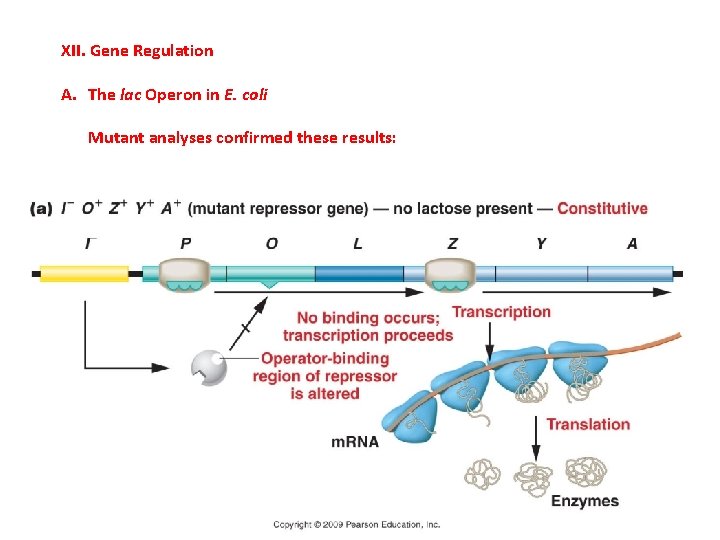
XII. Gene Regulation A. The lac Operon in E. coli Mutant analyses confirmed these results:
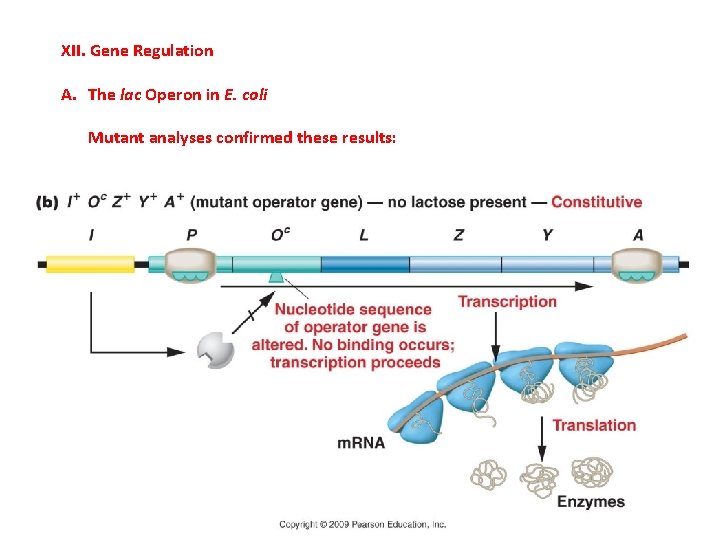
XII. Gene Regulation A. The lac Operon in E. coli Mutant analyses confirmed these results:
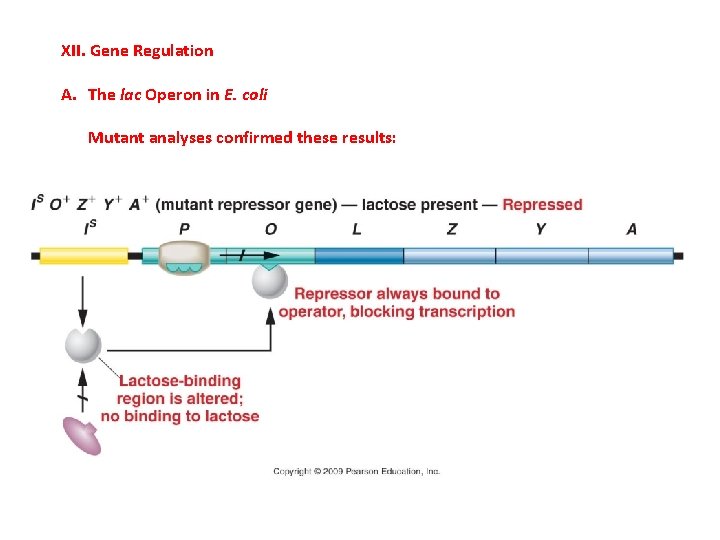
XII. Gene Regulation A. The lac Operon in E. coli Mutant analyses confirmed these results:
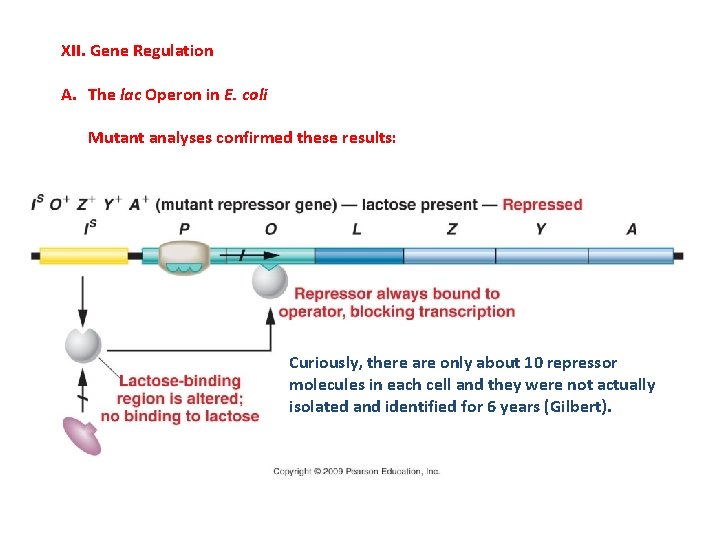
XII. Gene Regulation A. The lac Operon in E. coli Mutant analyses confirmed these results: Curiously, there are only about 10 repressor molecules in each cell and they were not actually isolated and identified for 6 years (Gilbert).
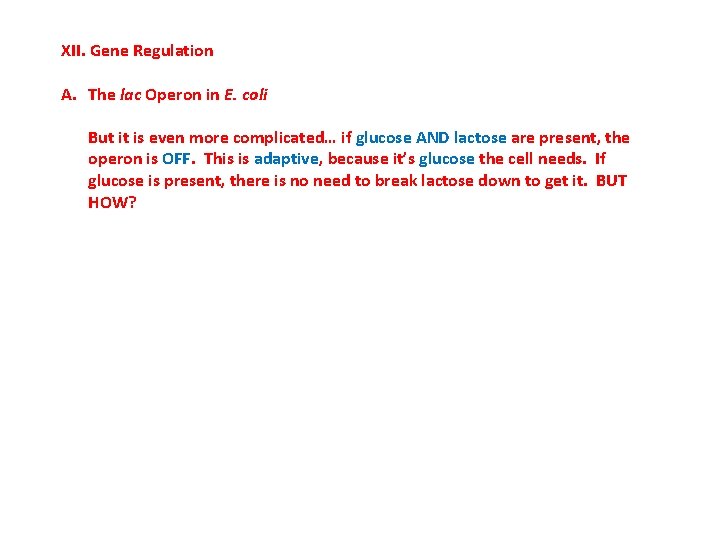
XII. Gene Regulation A. The lac Operon in E. coli But it is even more complicated… if glucose AND lactose are present, the operon is OFF. This is adaptive, because it’s glucose the cell needs. If glucose is present, there is no need to break lactose down to get it. BUT HOW?
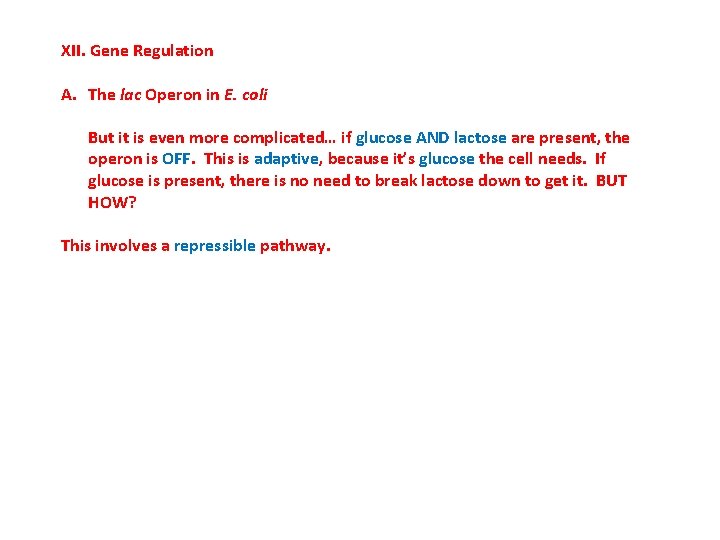
XII. Gene Regulation A. The lac Operon in E. coli But it is even more complicated… if glucose AND lactose are present, the operon is OFF. This is adaptive, because it’s glucose the cell needs. If glucose is present, there is no need to break lactose down to get it. BUT HOW? This involves a repressible pathway.
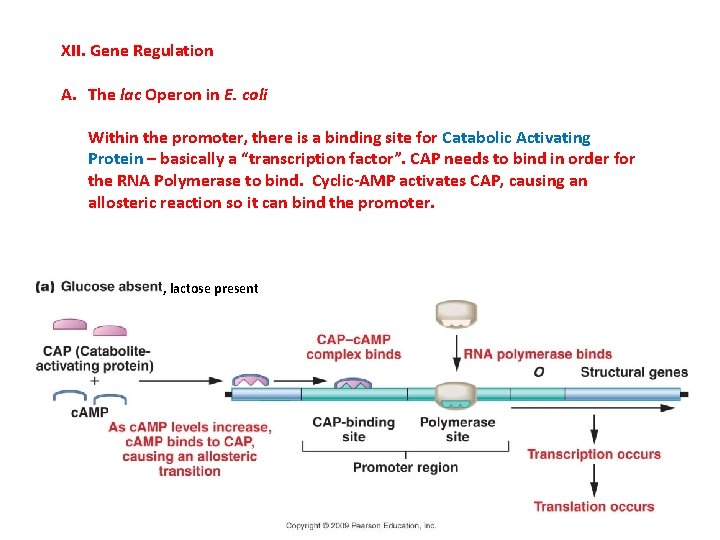
XII. Gene Regulation A. The lac Operon in E. coli Within the promoter, there is a binding site for Catabolic Activating Protein – basically a “transcription factor”. CAP needs to bind in order for the RNA Polymerase to bind. Cyclic-AMP activates CAP, causing an allosteric reaction so it can bind the promoter. , lactose present
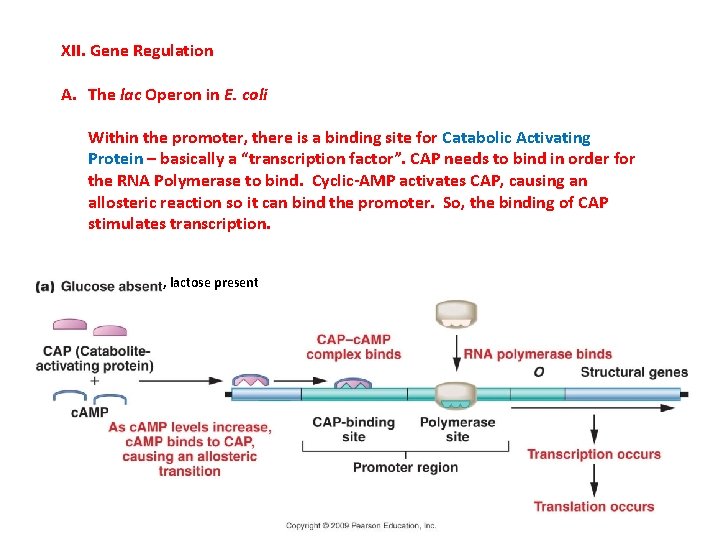
XII. Gene Regulation A. The lac Operon in E. coli Within the promoter, there is a binding site for Catabolic Activating Protein – basically a “transcription factor”. CAP needs to bind in order for the RNA Polymerase to bind. Cyclic-AMP activates CAP, causing an allosteric reaction so it can bind the promoter. So, the binding of CAP stimulates transcription. , lactose present
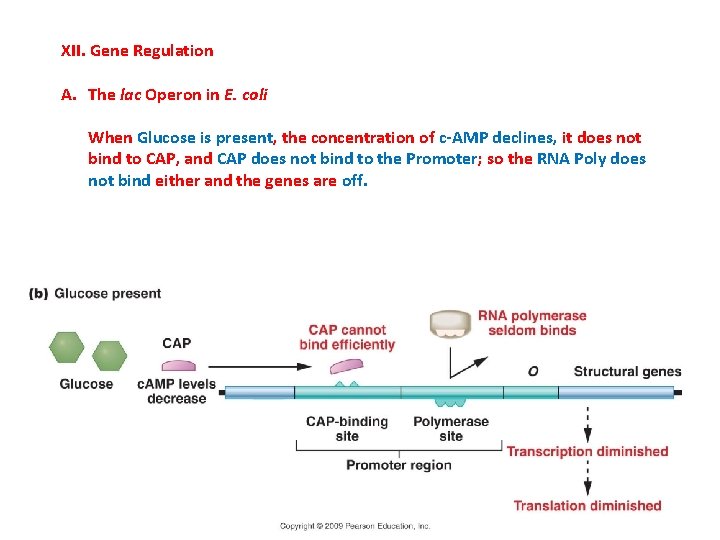
XII. Gene Regulation A. The lac Operon in E. coli When Glucose is present, the concentration of c-AMP declines, it does not bind to CAP, and CAP does not bind to the Promoter; so the RNA Poly does not bind either and the genes are off. , lactose present
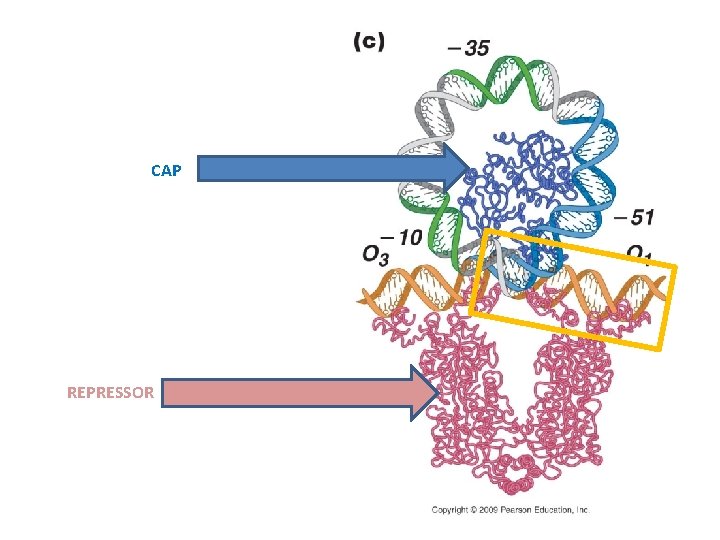
CAP REPRESSOR
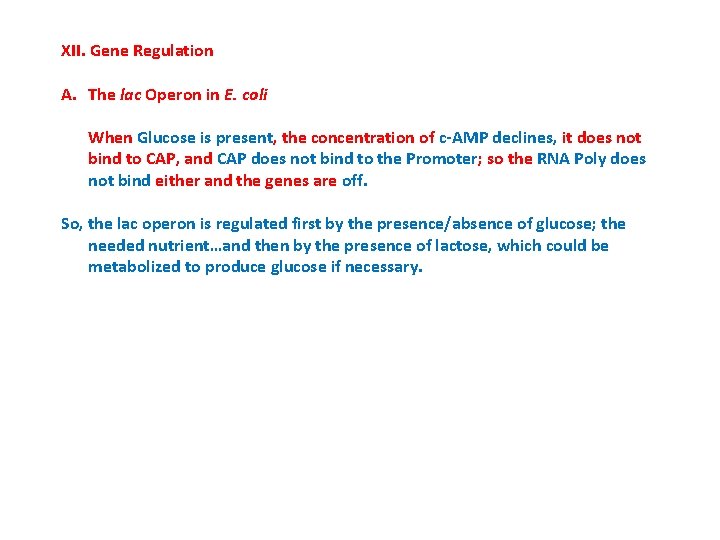
XII. Gene Regulation A. The lac Operon in E. coli When Glucose is present, the concentration of c-AMP declines, it does not bind to CAP, and CAP does not bind to the Promoter; so the RNA Poly does not bind either and the genes are off. So, the lac operon is regulated first by the presence/absence of glucose; the needed nutrient…and then by the presence of lactose, which could be metabolized to produce glucose if necessary.
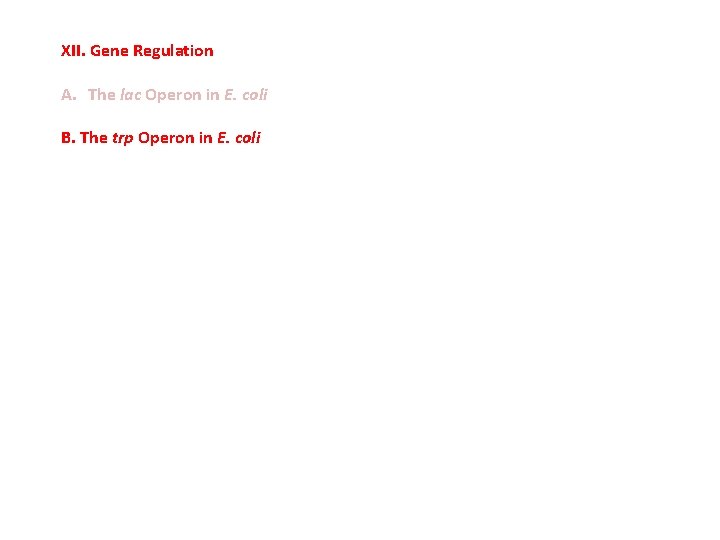
XII. Gene Regulation A. The lac Operon in E. coli B. The trp Operon in E. coli
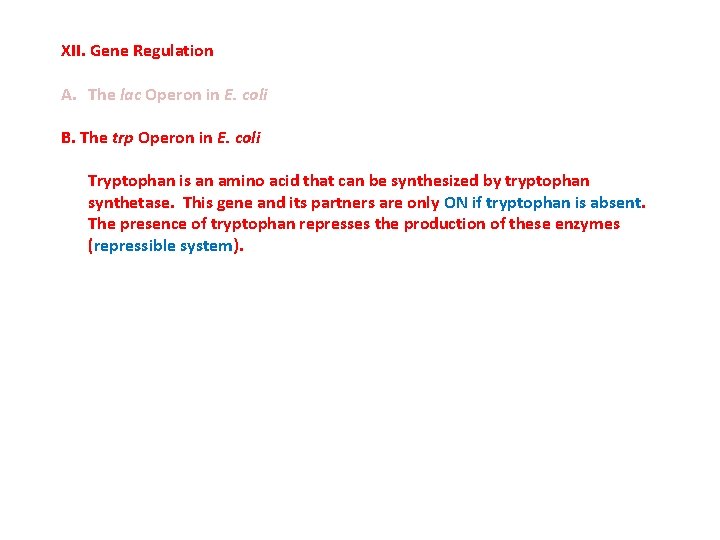
XII. Gene Regulation A. The lac Operon in E. coli B. The trp Operon in E. coli Tryptophan is an amino acid that can be synthesized by tryptophan synthetase. This gene and its partners are only ON if tryptophan is absent. The presence of tryptophan represses the production of these enzymes (repressible system).
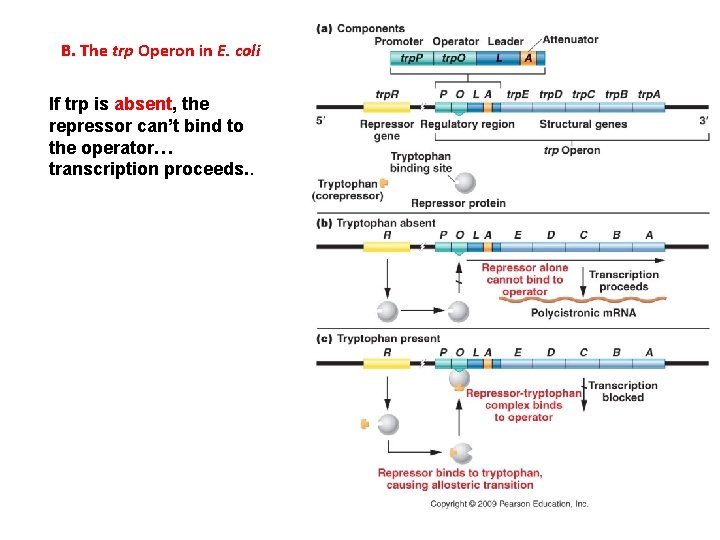
B. The trp Operon in E. coli If trp is absent, the repressor can’t bind to the operator… transcription proceeds. .
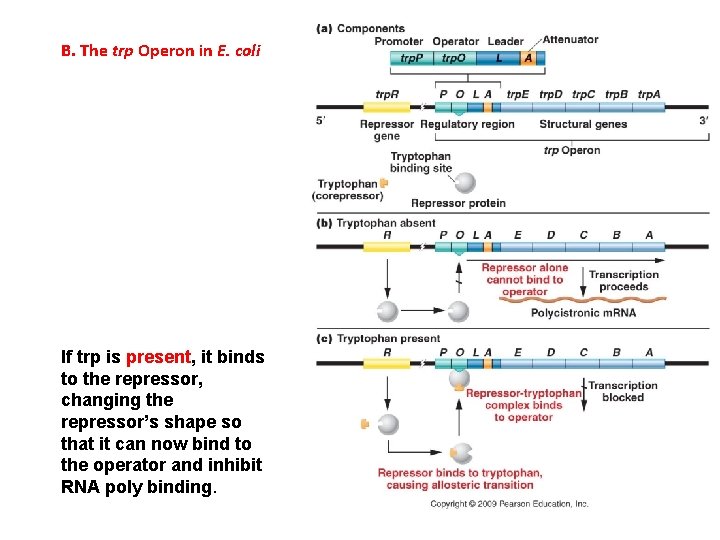
B. The trp Operon in E. coli If trp is present, it binds to the repressor, changing the repressor’s shape so that it can now bind to the operator and inhibit RNA poly binding.
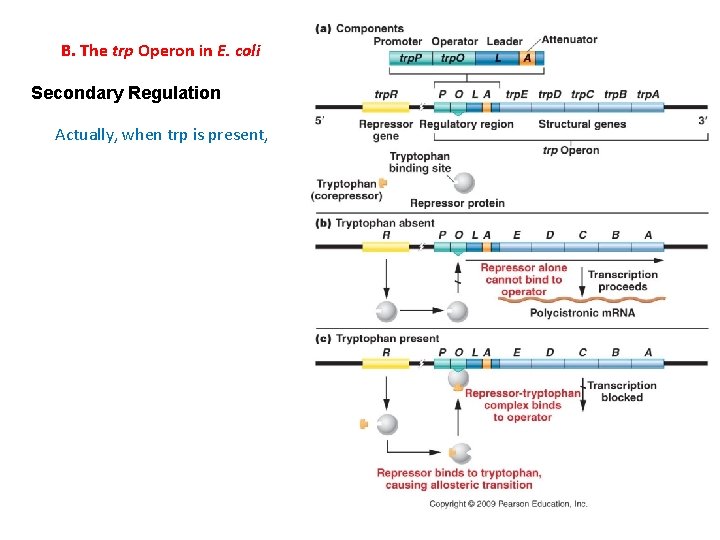
B. The trp Operon in E. coli Secondary Regulation Actually, when trp is present,
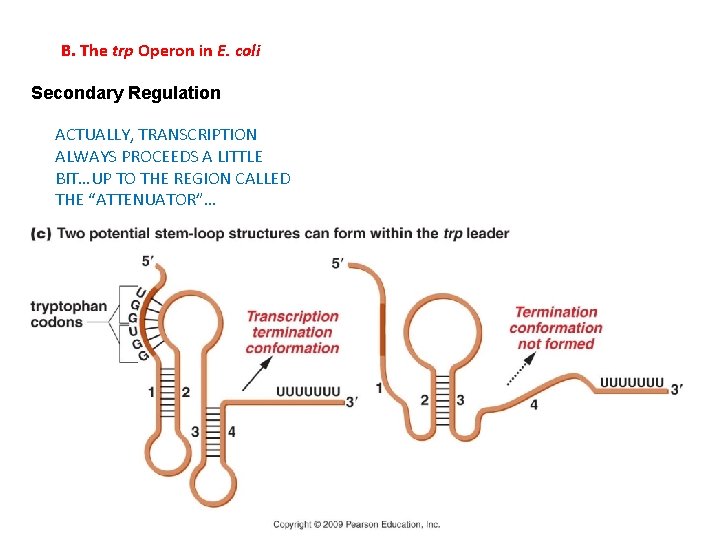
B. The trp Operon in E. coli Secondary Regulation ACTUALLY, TRANSCRIPTION ALWAYS PROCEEDS A LITTLE BIT…UP TO THE REGION CALLED THE “ATTENUATOR”…
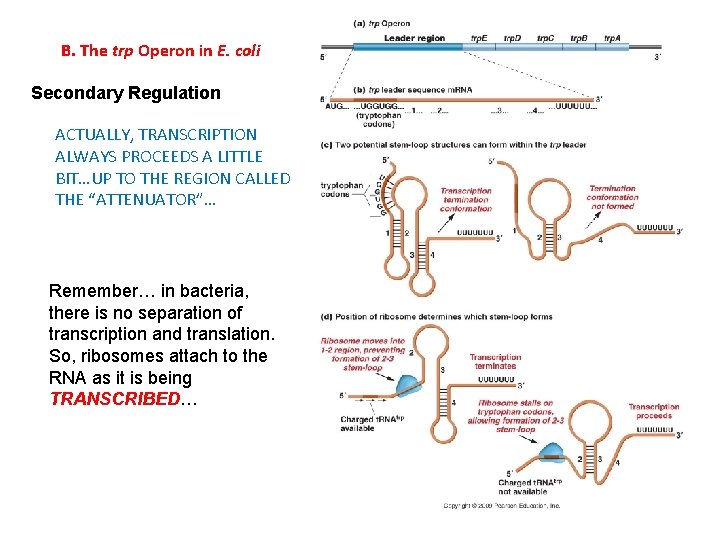
B. The trp Operon in E. coli Secondary Regulation ACTUALLY, TRANSCRIPTION ALWAYS PROCEEDS A LITTLE BIT…UP TO THE REGION CALLED THE “ATTENUATOR”… Remember… in bacteria, there is no separation of transcription and translation. So, ribosomes attach to the RNA as it is being TRANSCRIBED…
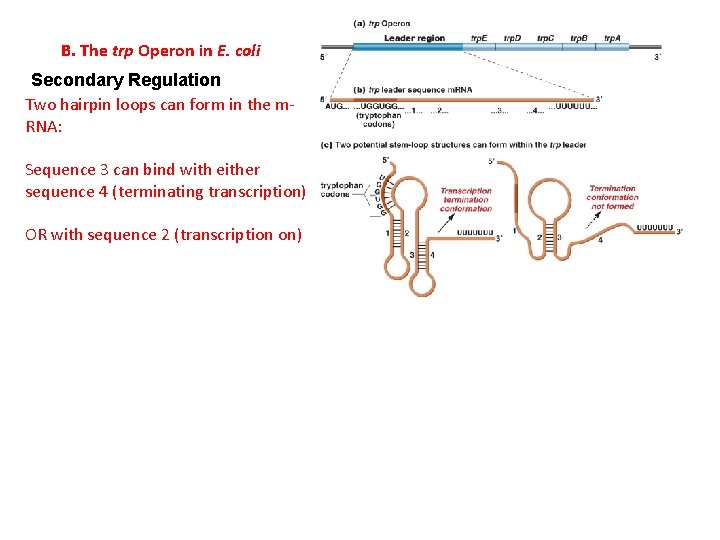
B. The trp Operon in E. coli Secondary Regulation Two hairpin loops can form in the m. RNA: Sequence 3 can bind with either sequence 4 (terminating transcription) OR with sequence 2 (transcription on)
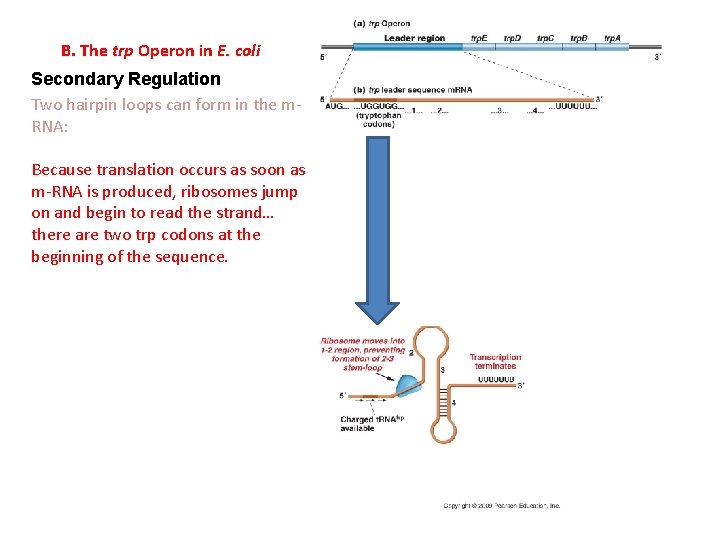
B. The trp Operon in E. coli Secondary Regulation Two hairpin loops can form in the m. RNA: Because translation occurs as soon as m-RNA is produced, ribosomes jump on and begin to read the strand… there are two trp codons at the beginning of the sequence.
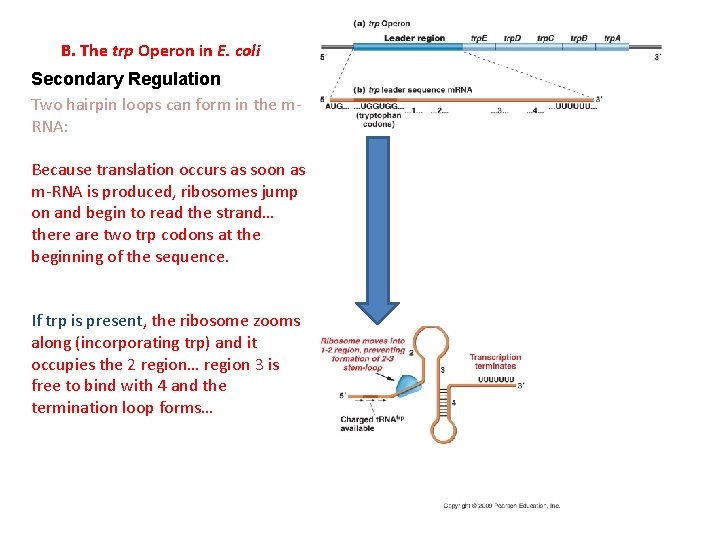
B. The trp Operon in E. coli Secondary Regulation Two hairpin loops can form in the m. RNA: Because translation occurs as soon as m-RNA is produced, ribosomes jump on and begin to read the strand… there are two trp codons at the beginning of the sequence. If trp is present, the ribosome zooms along (incorporating trp) and it occupies the 2 region… region 3 is free to bind with 4 and the termination loop forms…
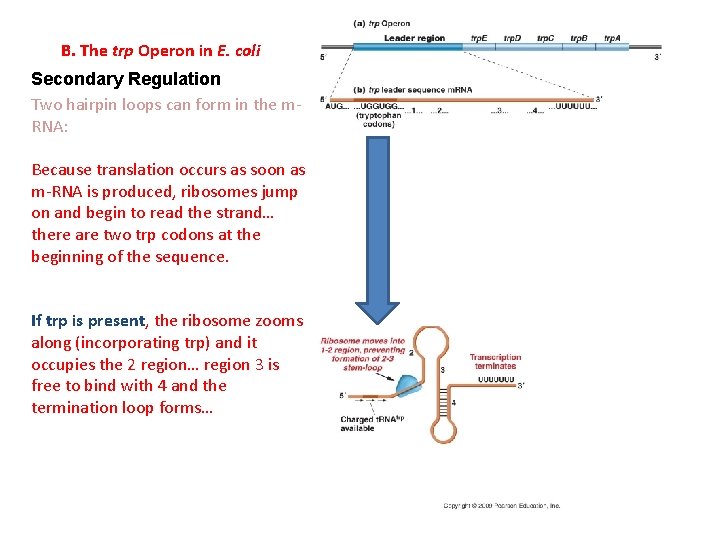
B. The trp Operon in E. coli Secondary Regulation Two hairpin loops can form in the m. RNA: Because translation occurs as soon as m-RNA is produced, ribosomes jump on and begin to read the strand… there are two trp codons at the beginning of the sequence. If trp is present, the ribosome zooms along (incorporating trp) and it occupies the 2 region… region 3 is free to bind with 4 and the termination loop forms…
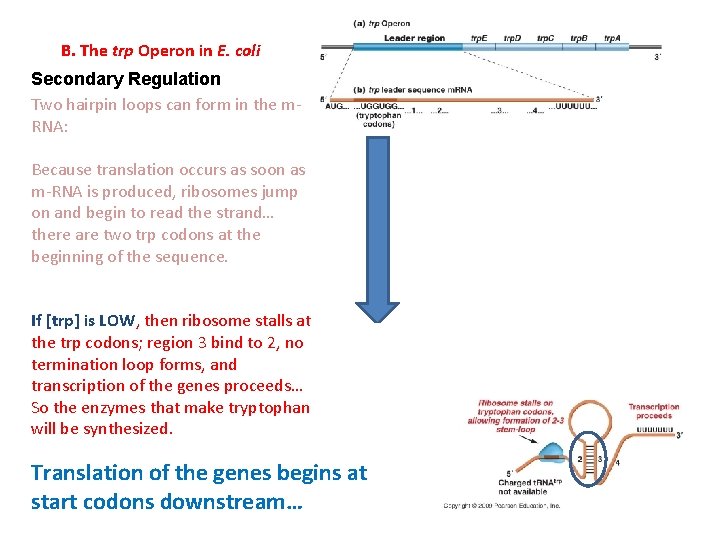
B. The trp Operon in E. coli Secondary Regulation Two hairpin loops can form in the m. RNA: Because translation occurs as soon as m-RNA is produced, ribosomes jump on and begin to read the strand… there are two trp codons at the beginning of the sequence. If [trp] is LOW, then ribosome stalls at the trp codons; region 3 bind to 2, no termination loop forms, and transcription of the genes proceeds… So the enzymes that make tryptophan will be synthesized. Translation of the genes begins at start codons downstream…
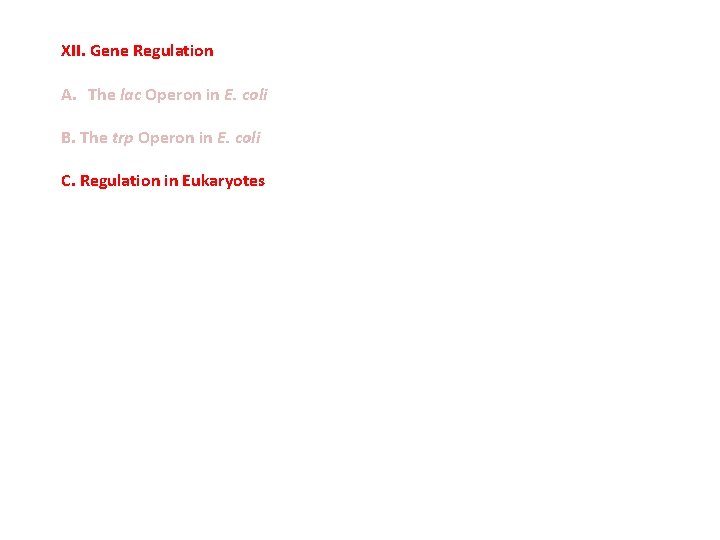
XII. Gene Regulation A. The lac Operon in E. coli B. The trp Operon in E. coli C. Regulation in Eukaryotes
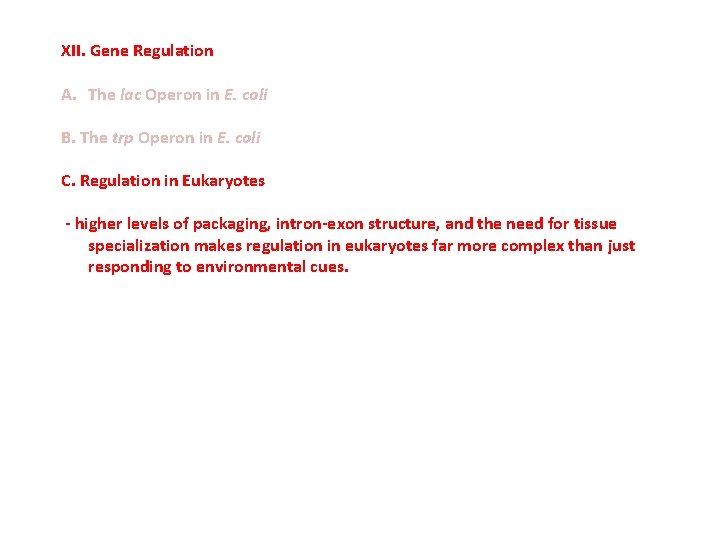
XII. Gene Regulation A. The lac Operon in E. coli B. The trp Operon in E. coli C. Regulation in Eukaryotes - higher levels of packaging, intron-exon structure, and the need for tissue specialization makes regulation in eukaryotes far more complex than just responding to environmental cues.
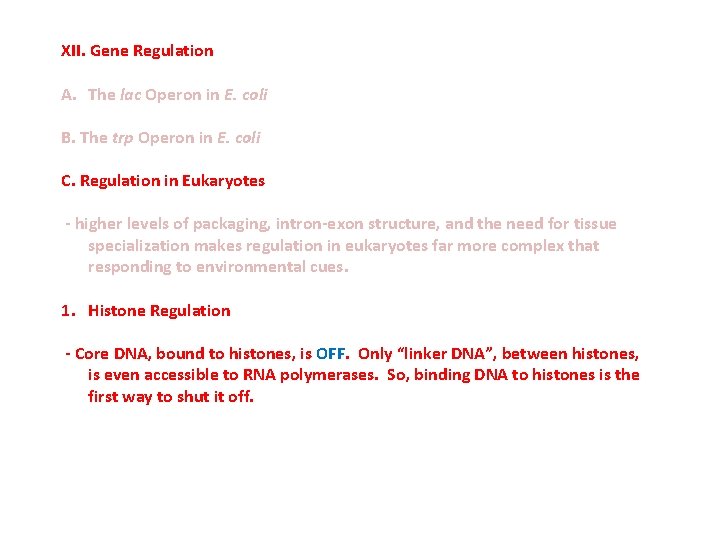
XII. Gene Regulation A. The lac Operon in E. coli B. The trp Operon in E. coli C. Regulation in Eukaryotes - higher levels of packaging, intron-exon structure, and the need for tissue specialization makes regulation in eukaryotes far more complex that responding to environmental cues. 1. Histone Regulation - Core DNA, bound to histones, is OFF. Only “linker DNA”, between histones, is even accessible to RNA polymerases. So, binding DNA to histones is the first way to shut it off.
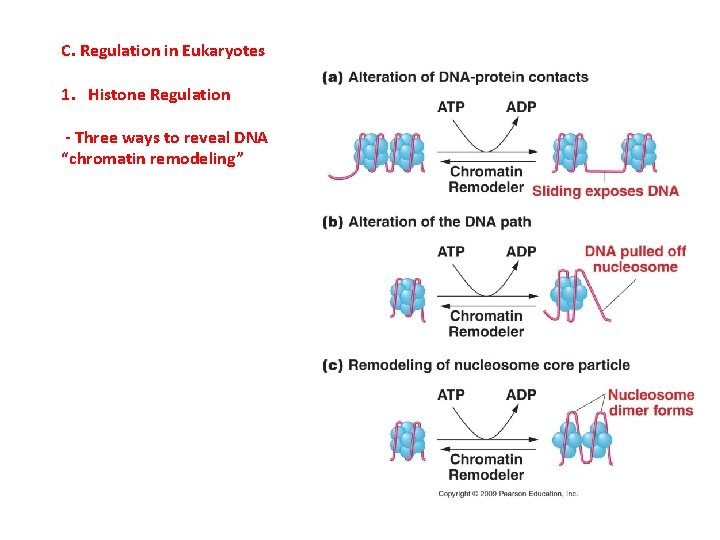
C. Regulation in Eukaryotes 1. Histone Regulation - Three ways to reveal DNA “chromatin remodeling”
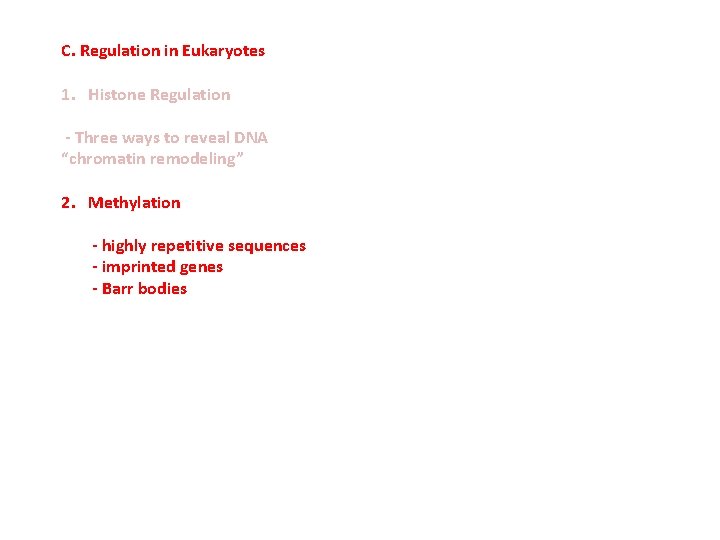
C. Regulation in Eukaryotes 1. Histone Regulation - Three ways to reveal DNA “chromatin remodeling” 2. Methylation - highly repetitive sequences - imprinted genes - Barr bodies
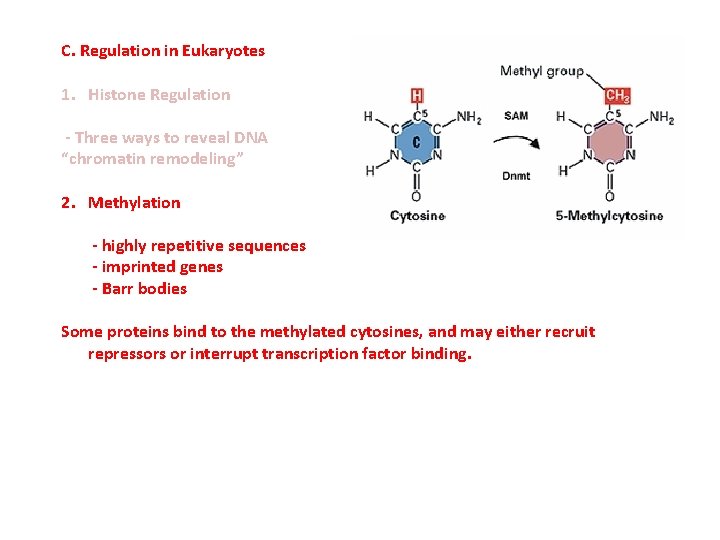
C. Regulation in Eukaryotes 1. Histone Regulation - Three ways to reveal DNA “chromatin remodeling” 2. Methylation - highly repetitive sequences - imprinted genes - Barr bodies Some proteins bind to the methylated cytosines, and may either recruit repressors or interrupt transcription factor binding.
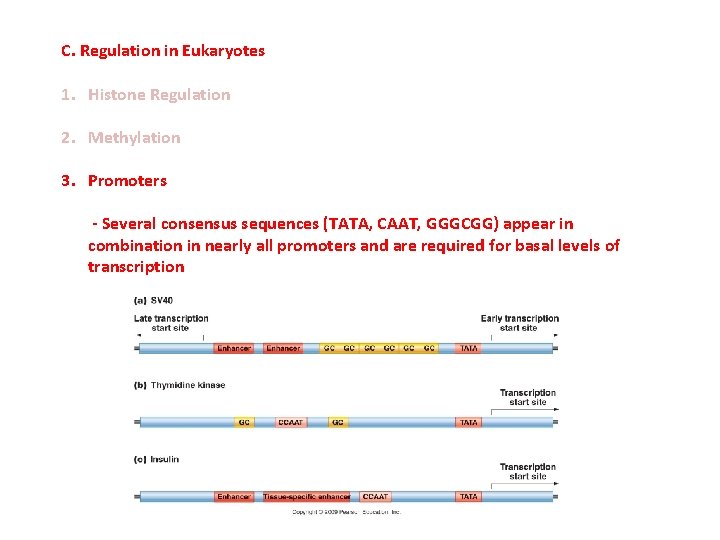
C. Regulation in Eukaryotes 1. Histone Regulation 2. Methylation 3. Promoters - Several consensus sequences (TATA, CAAT, GGGCGG) appear in combination in nearly all promoters and are required for basal levels of transcription
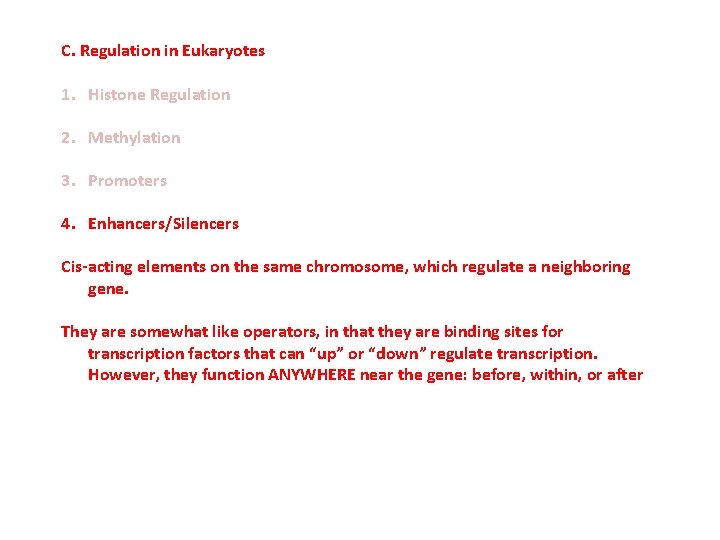
C. Regulation in Eukaryotes 1. Histone Regulation 2. Methylation 3. Promoters 4. Enhancers/Silencers Cis-acting elements on the same chromosome, which regulate a neighboring gene. They are somewhat like operators, in that they are binding sites for transcription factors that can “up” or “down” regulate transcription. However, they function ANYWHERE near the gene: before, within, or after
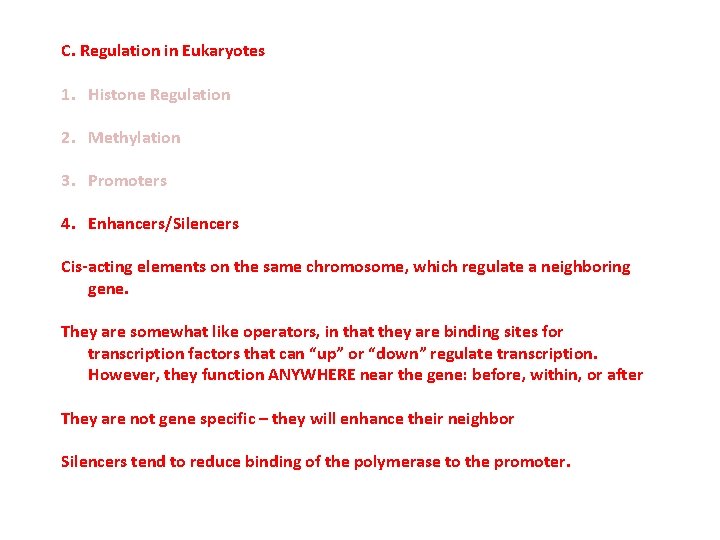
C. Regulation in Eukaryotes 1. Histone Regulation 2. Methylation 3. Promoters 4. Enhancers/Silencers Cis-acting elements on the same chromosome, which regulate a neighboring gene. They are somewhat like operators, in that they are binding sites for transcription factors that can “up” or “down” regulate transcription. However, they function ANYWHERE near the gene: before, within, or after They are not gene specific – they will enhance their neighbor Silencers tend to reduce binding of the polymerase to the promoter.
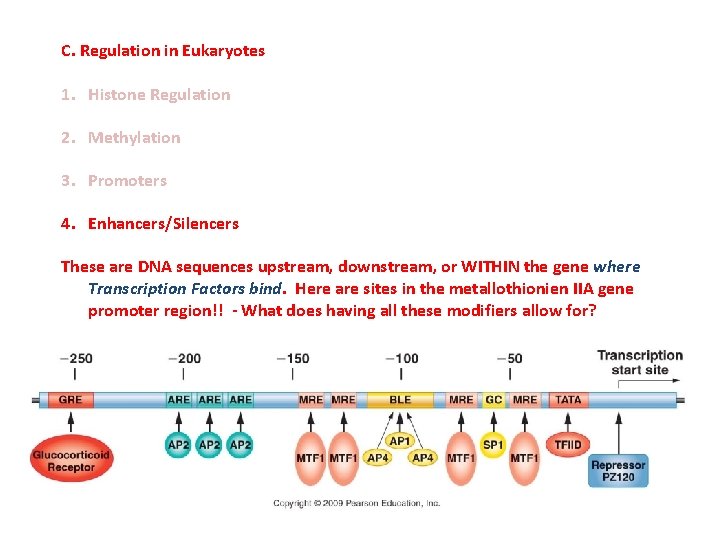
C. Regulation in Eukaryotes 1. Histone Regulation 2. Methylation 3. Promoters 4. Enhancers/Silencers These are DNA sequences upstream, downstream, or WITHIN the gene where Transcription Factors bind. Here are sites in the metallothionien IIA gene promoter region!! - What does having all these modifiers allow for?
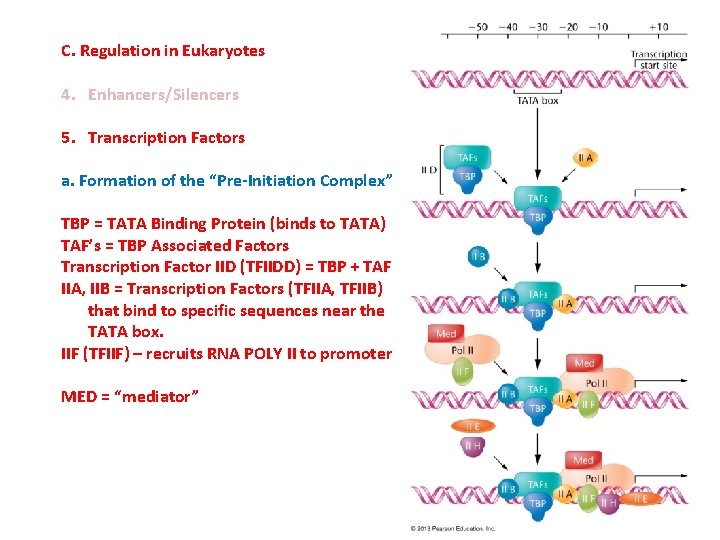
C. Regulation in Eukaryotes 4. Enhancers/Silencers 5. Transcription Factors a. Formation of the “Pre-Initiation Complex” TBP = TATA Binding Protein (binds to TATA) TAF’s = TBP Associated Factors Transcription Factor IID (TFIIDD) = TBP + TAF IIA, IIB = Transcription Factors (TFIIA, TFIIB) that bind to specific sequences near the TATA box. IIF (TFIIF) – recruits RNA POLY II to promoter MED = “mediator”
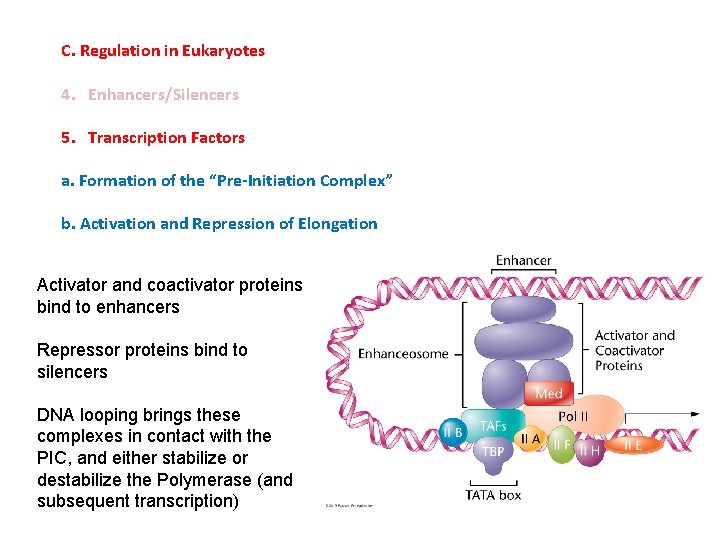
C. Regulation in Eukaryotes 4. Enhancers/Silencers 5. Transcription Factors a. Formation of the “Pre-Initiation Complex” b. Activation and Repression of Elongation Activator and coactivator proteins bind to enhancers Repressor proteins bind to silencers DNA looping brings these complexes in contact with the PIC, and either stabilize or destabilize the Polymerase (and subsequent transcription)
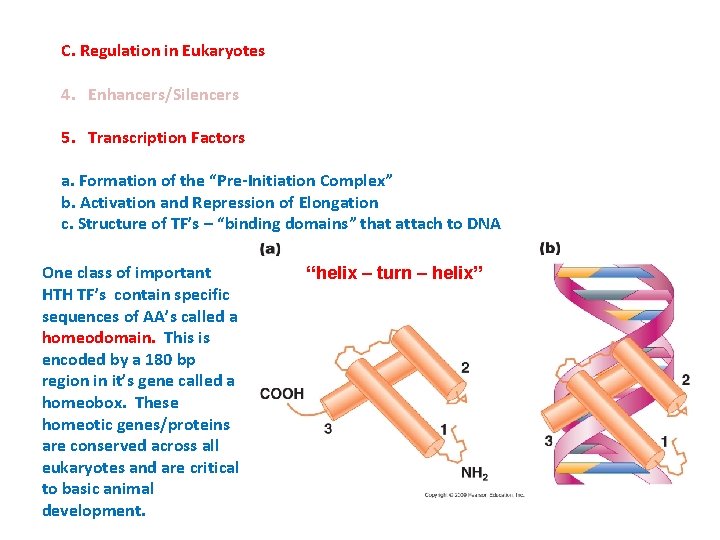
C. Regulation in Eukaryotes 4. Enhancers/Silencers 5. Transcription Factors a. Formation of the “Pre-Initiation Complex” b. Activation and Repression of Elongation c. Structure of TF’s – “binding domains” that attach to DNA One class of important HTH TF’s contain specific sequences of AA’s called a homeodomain. This is encoded by a 180 bp region in it’s gene called a homeobox. These homeotic genes/proteins are conserved across all eukaryotes and are critical to basic animal development. “helix – turn – helix”
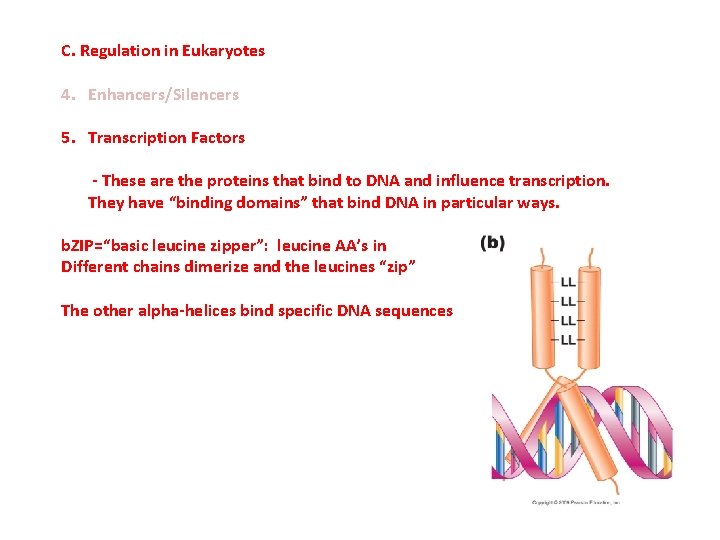
C. Regulation in Eukaryotes 4. Enhancers/Silencers 5. Transcription Factors - These are the proteins that bind to DNA and influence transcription. They have “binding domains” that bind DNA in particular ways. b. ZIP=“basic leucine zipper”: leucine AA’s in Different chains dimerize and the leucines “zip” The other alpha-helices bind specific DNA sequences
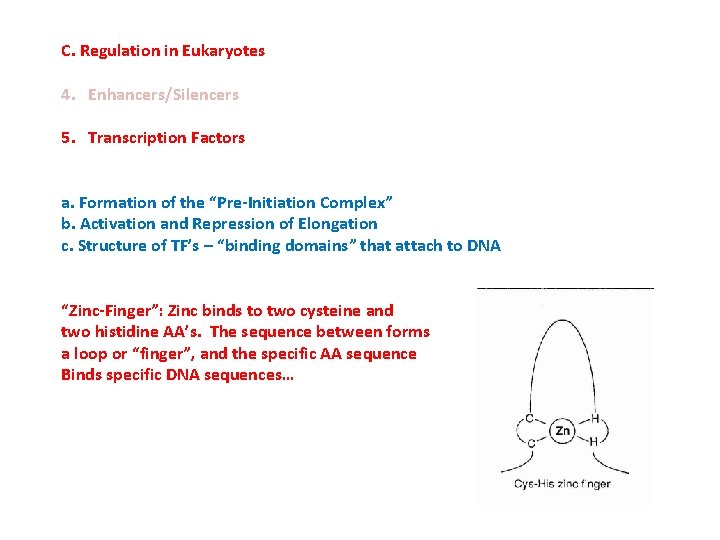
C. Regulation in Eukaryotes 4. Enhancers/Silencers 5. Transcription Factors a. Formation of the “Pre-Initiation Complex” b. Activation and Repression of Elongation c. Structure of TF’s – “binding domains” that attach to DNA “Zinc-Finger”: Zinc binds to two cysteine and two histidine AA’s. The sequence between forms a loop or “finger”, and the specific AA sequence Binds specific DNA sequences…
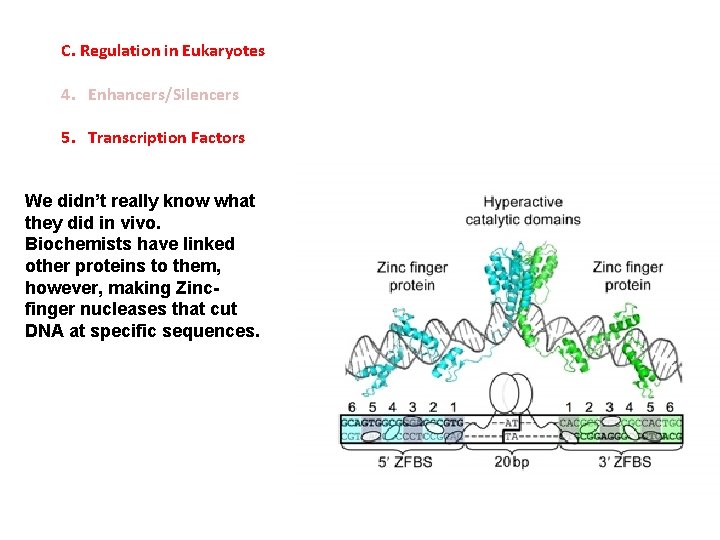
C. Regulation in Eukaryotes 4. Enhancers/Silencers 5. Transcription Factors We didn’t really know what they did in vivo. Biochemists have linked other proteins to them, however, making Zincfinger nucleases that cut DNA at specific sequences.
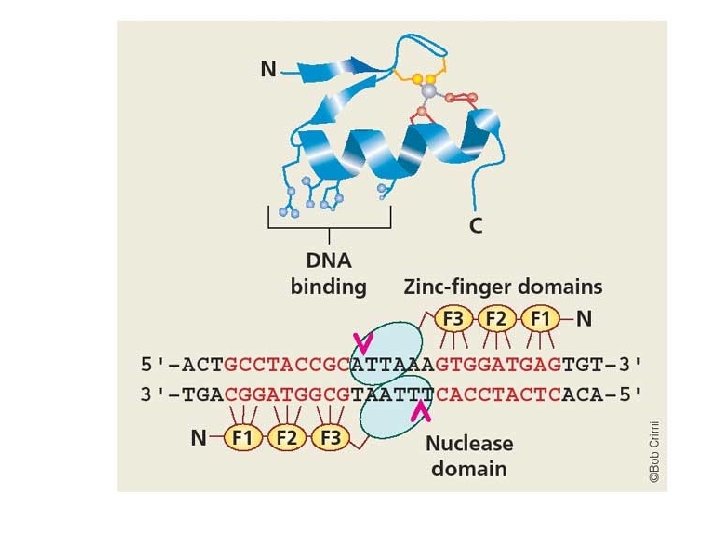
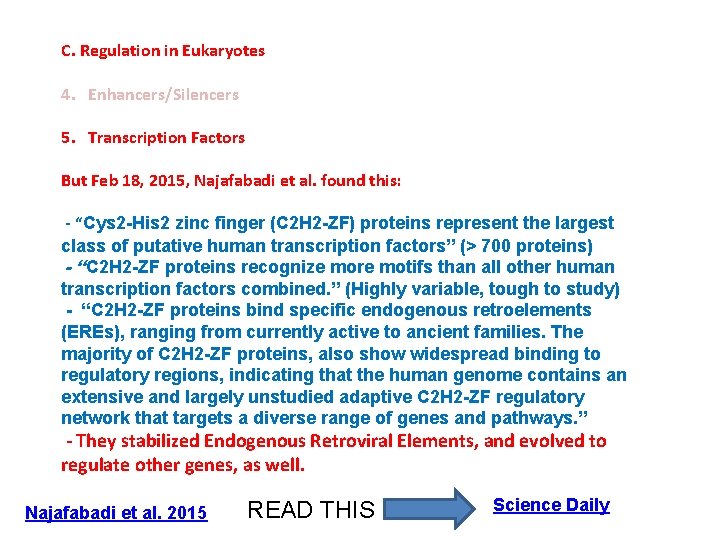
C. Regulation in Eukaryotes 4. Enhancers/Silencers 5. Transcription Factors But Feb 18, 2015, Najafabadi et al. found this: - “Cys 2 -His 2 zinc finger (C 2 H 2 -ZF) proteins represent the largest class of putative human transcription factors” (> 700 proteins) - “C 2 H 2 -ZF proteins recognize more motifs than all other human transcription factors combined. ” (Highly variable, tough to study) - “C 2 H 2 -ZF proteins bind specific endogenous retroelements (EREs), ranging from currently active to ancient families. The majority of C 2 H 2 -ZF proteins, also show widespread binding to regulatory regions, indicating that the human genome contains an extensive and largely unstudied adaptive C 2 H 2 -ZF regulatory network that targets a diverse range of genes and pathways. ” - They stabilized Endogenous Retroviral Elements, and evolved to regulate other genes, as well. Najafabadi et al. 2015 READ THIS Science Daily
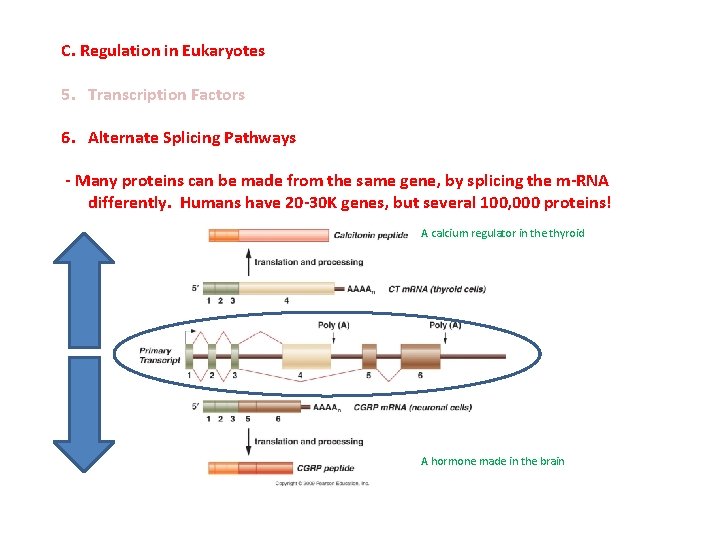
C. Regulation in Eukaryotes 5. Transcription Factors 6. Alternate Splicing Pathways - Many proteins can be made from the same gene, by splicing the m-RNA differently. Humans have 20 -30 K genes, but several 100, 000 proteins! A calcium regulator in the thyroid A hormone made in the brain
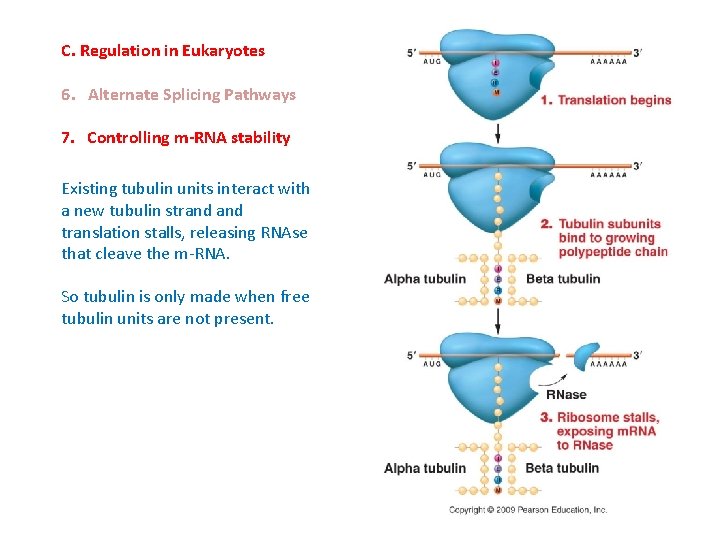
C. Regulation in Eukaryotes 6. Alternate Splicing Pathways 7. Controlling m-RNA stability Existing tubulin units interact with a new tubulin strand translation stalls, releasing RNAse that cleave the m-RNA. So tubulin is only made when free tubulin units are not present.
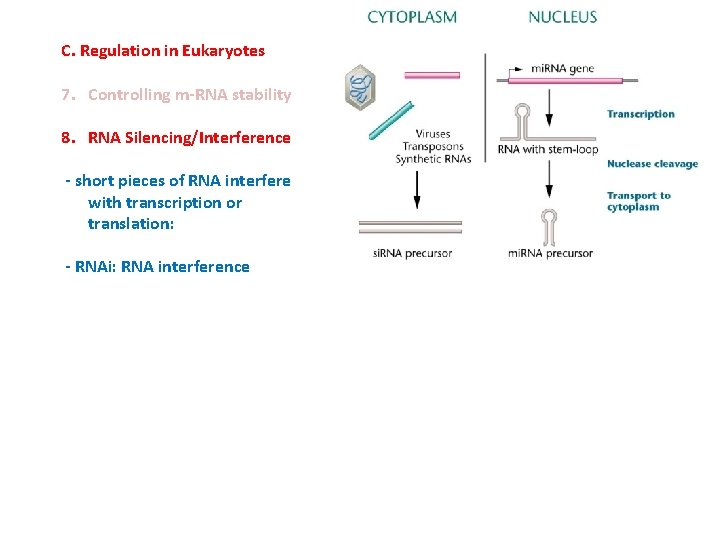
C. Regulation in Eukaryotes 7. Controlling m-RNA stability 8. RNA Silencing/Interference - short pieces of RNA interfere with transcription or translation: - RNAi: RNA interference
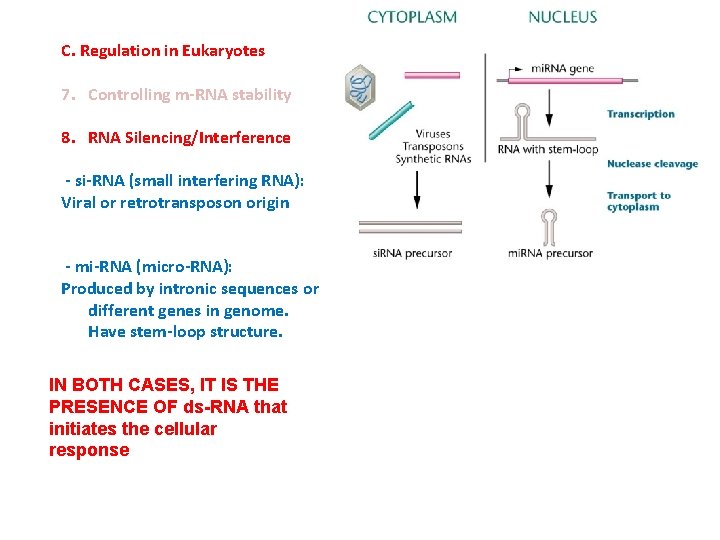
C. Regulation in Eukaryotes 7. Controlling m-RNA stability 8. RNA Silencing/Interference - si-RNA (small interfering RNA): Viral or retrotransposon origin - mi-RNA (micro-RNA): Produced by intronic sequences or different genes in genome. Have stem-loop structure. IN BOTH CASES, IT IS THE PRESENCE OF ds-RNA that initiates the cellular response
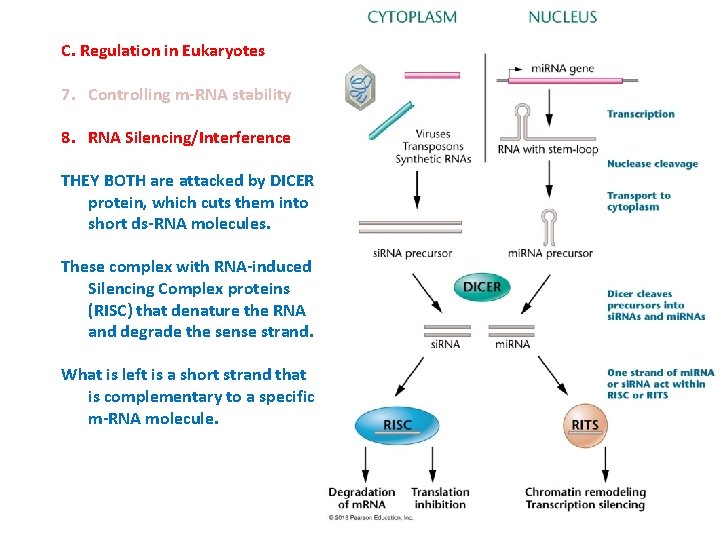
C. Regulation in Eukaryotes 7. Controlling m-RNA stability 8. RNA Silencing/Interference THEY BOTH are attacked by DICER protein, which cuts them into short ds-RNA molecules. These complex with RNA-induced Silencing Complex proteins (RISC) that denature the RNA and degrade the sense strand. What is left is a short strand that is complementary to a specific m-RNA molecule.
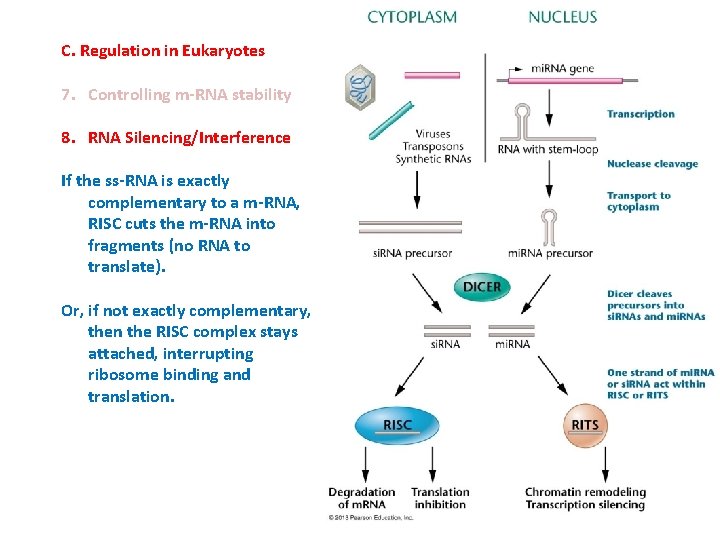
C. Regulation in Eukaryotes 7. Controlling m-RNA stability 8. RNA Silencing/Interference If the ss-RNA is exactly complementary to a m-RNA, RISC cuts the m-RNA into fragments (no RNA to translate). Or, if not exactly complementary, then the RISC complex stays attached, interrupting ribosome binding and translation.
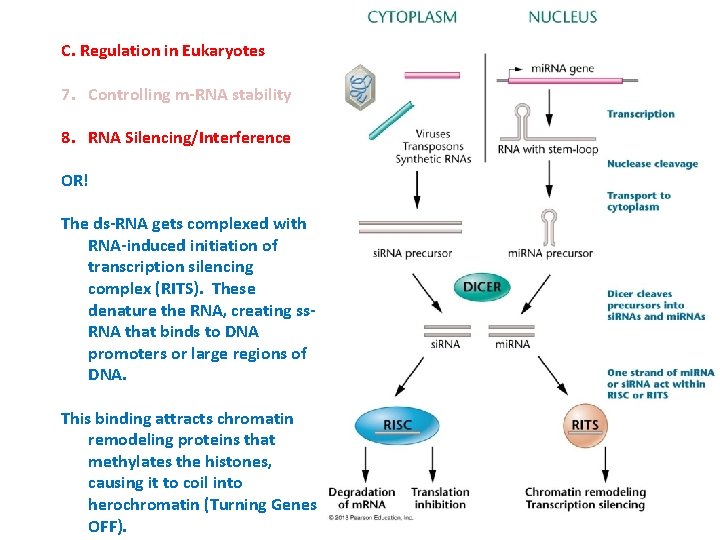
C. Regulation in Eukaryotes 7. Controlling m-RNA stability 8. RNA Silencing/Interference OR! The ds-RNA gets complexed with RNA-induced initiation of transcription silencing complex (RITS). These denature the RNA, creating ss. RNA that binds to DNA promoters or large regions of DNA. This binding attracts chromatin remodeling proteins that methylates the histones, causing it to coil into herochromatin (Turning Genes OFF).
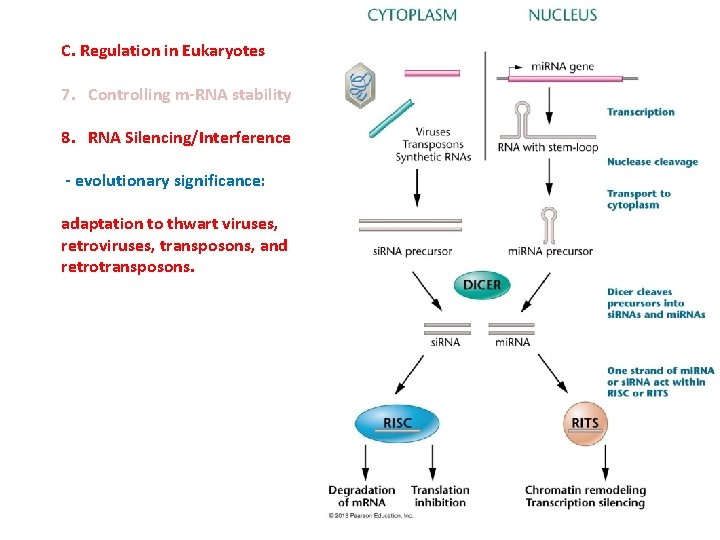
C. Regulation in Eukaryotes 7. Controlling m-RNA stability 8. RNA Silencing/Interference - evolutionary significance: adaptation to thwart viruses, retroviruses, transposons, and retrotransposons.
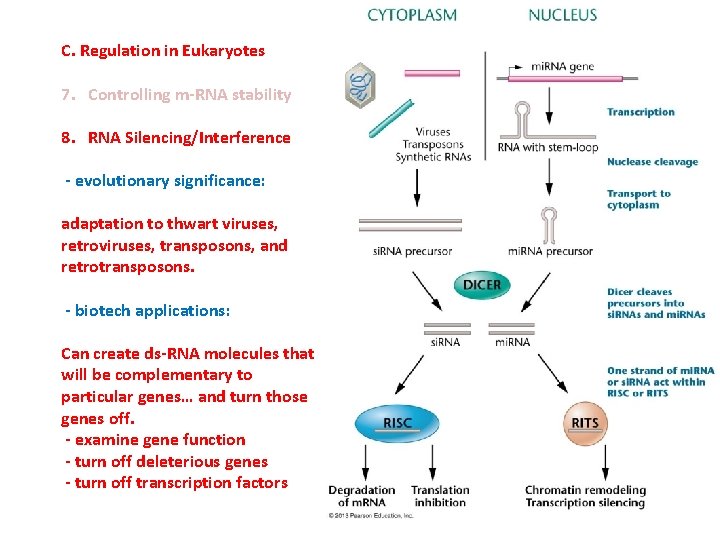
C. Regulation in Eukaryotes 7. Controlling m-RNA stability 8. RNA Silencing/Interference - evolutionary significance: adaptation to thwart viruses, retroviruses, transposons, and retrotransposons. - biotech applications: Can create ds-RNA molecules that will be complementary to particular genes… and turn those genes off. - examine gene function - turn off deleterious genes - turn off transcription factors
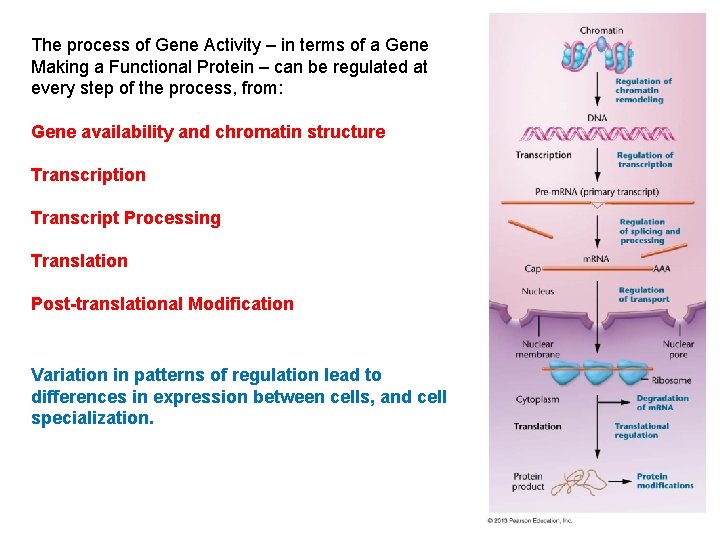
The process of Gene Activity – in terms of a Gene Making a Functional Protein – can be regulated at every step of the process, from: Gene availability and chromatin structure Transcription Transcript Processing Translation Post-translational Modification Variation in patterns of regulation lead to differences in expression between cells, and cell specialization.
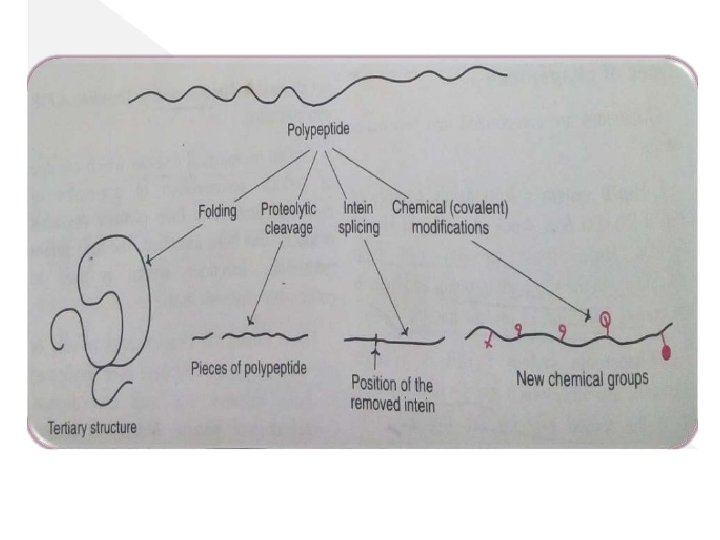
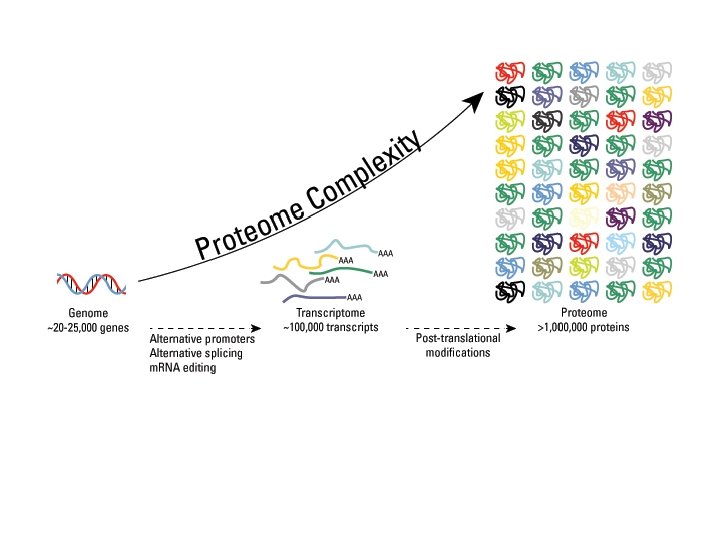
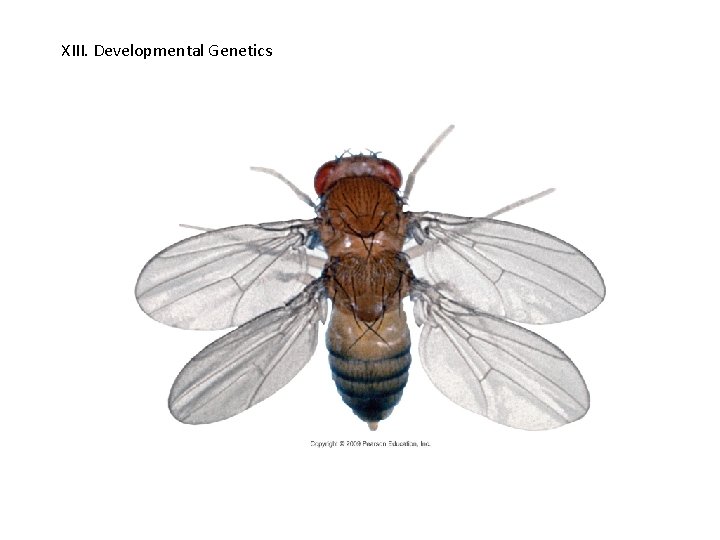
XIII. Developmental Genetics
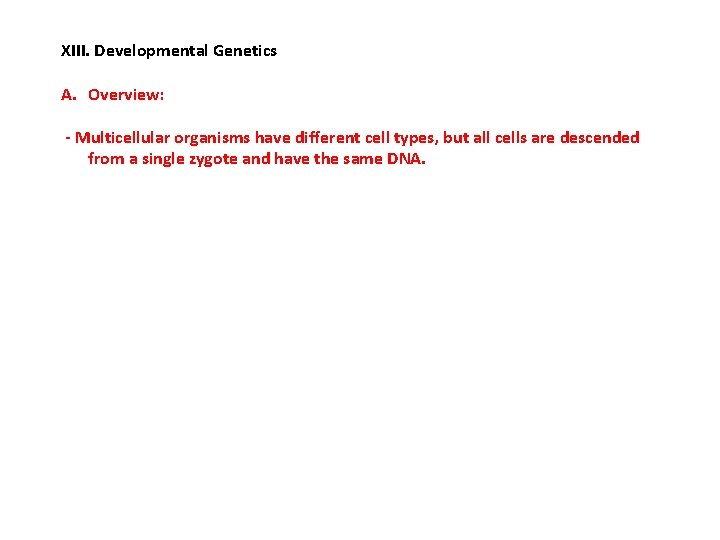
XIII. Developmental Genetics A. Overview: - Multicellular organisms have different cell types, but all cells are descended from a single zygote and have the same DNA.
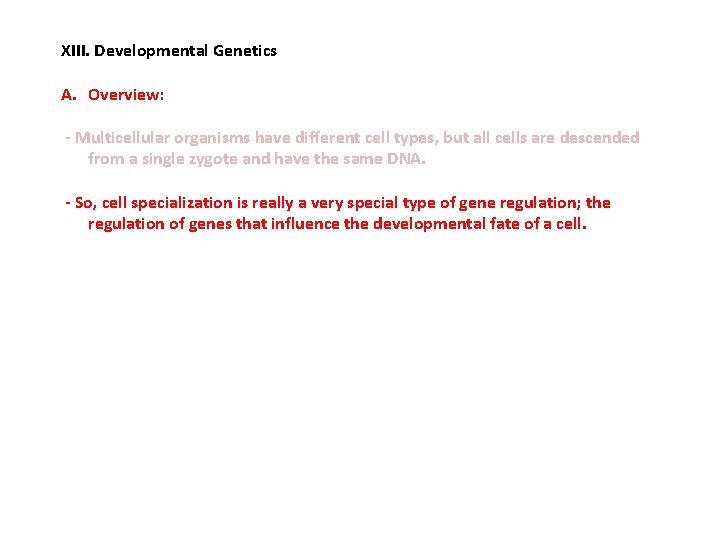
XIII. Developmental Genetics A. Overview: - Multicellular organisms have different cell types, but all cells are descended from a single zygote and have the same DNA. - So, cell specialization is really a very special type of gene regulation; the regulation of genes that influence the developmental fate of a cell.
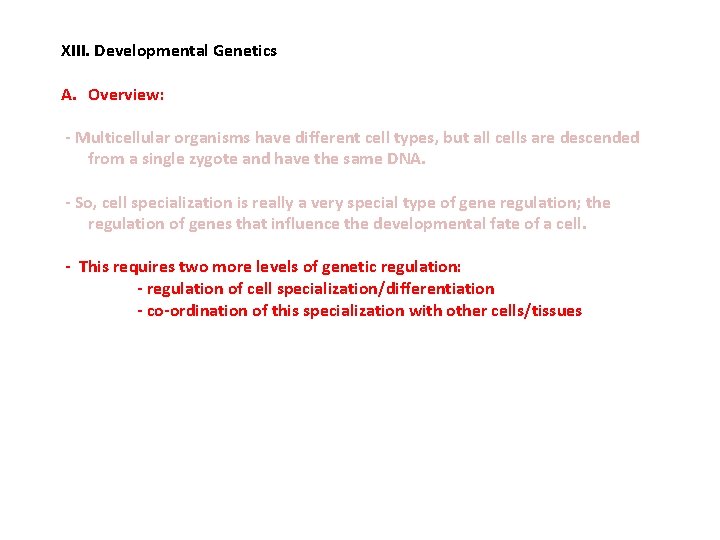
XIII. Developmental Genetics A. Overview: - Multicellular organisms have different cell types, but all cells are descended from a single zygote and have the same DNA. - So, cell specialization is really a very special type of gene regulation; the regulation of genes that influence the developmental fate of a cell. - This requires two more levels of genetic regulation: - regulation of cell specialization/differentiation - co-ordination of this specialization with other cells/tissues
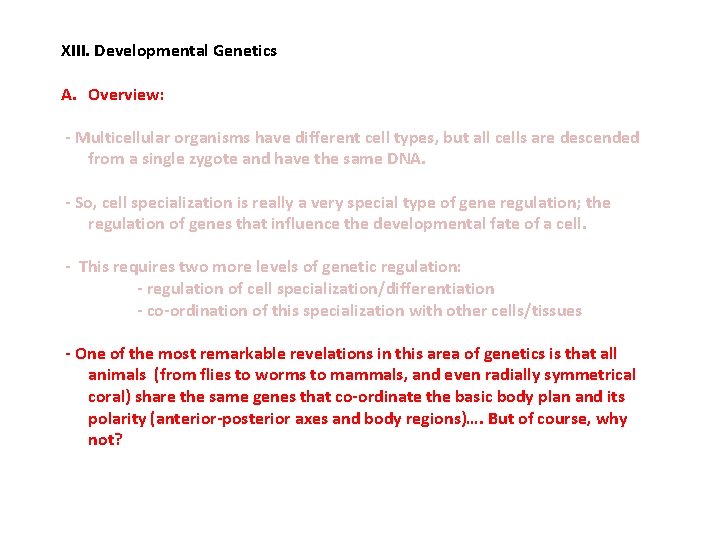
XIII. Developmental Genetics A. Overview: - Multicellular organisms have different cell types, but all cells are descended from a single zygote and have the same DNA. - So, cell specialization is really a very special type of gene regulation; the regulation of genes that influence the developmental fate of a cell. - This requires two more levels of genetic regulation: - regulation of cell specialization/differentiation - co-ordination of this specialization with other cells/tissues - One of the most remarkable revelations in this area of genetics is that all animals (from flies to worms to mammals, and even radially symmetrical coral) share the same genes that co-ordinate the basic body plan and its polarity (anterior-posterior axes and body regions)…. But of course, why not?
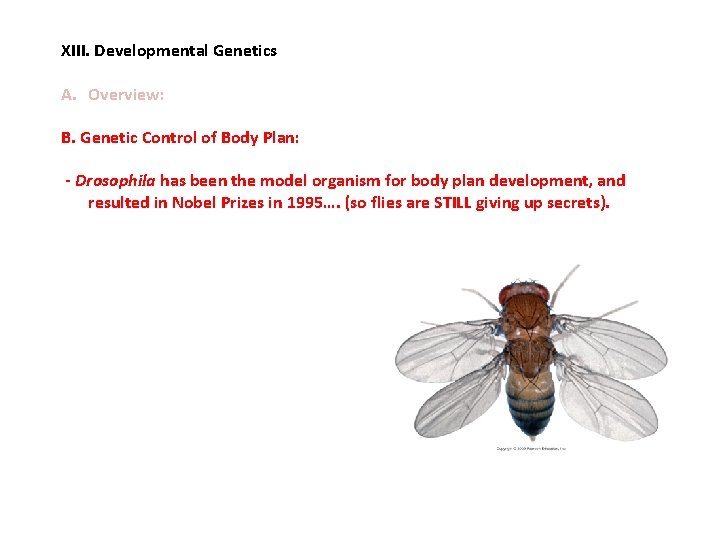
XIII. Developmental Genetics A. Overview: B. Genetic Control of Body Plan: - Drosophila has been the model organism for body plan development, and resulted in Nobel Prizes in 1995…. (so flies are STILL giving up secrets).
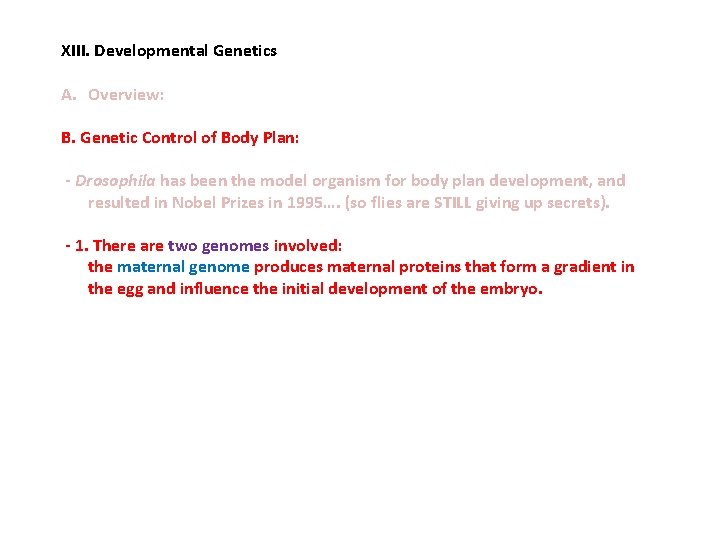
XIII. Developmental Genetics A. Overview: B. Genetic Control of Body Plan: - Drosophila has been the model organism for body plan development, and resulted in Nobel Prizes in 1995…. (so flies are STILL giving up secrets). - 1. There are two genomes involved: the maternal genome produces maternal proteins that form a gradient in the egg and influence the initial development of the embryo.
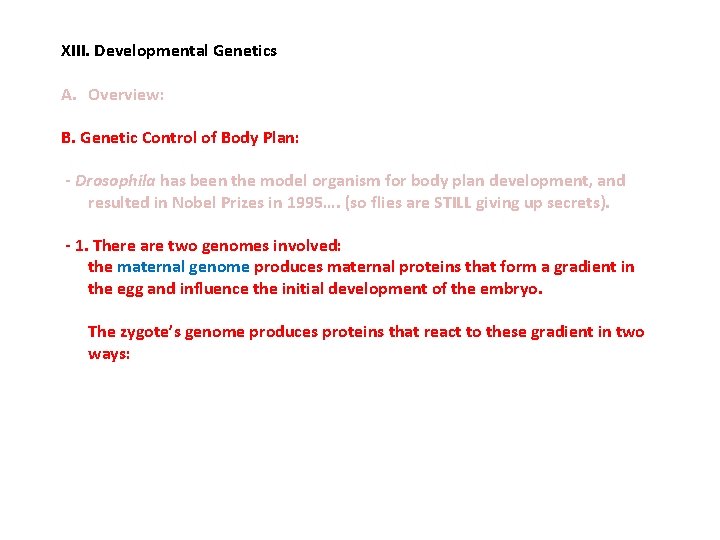
XIII. Developmental Genetics A. Overview: B. Genetic Control of Body Plan: - Drosophila has been the model organism for body plan development, and resulted in Nobel Prizes in 1995…. (so flies are STILL giving up secrets). - 1. There are two genomes involved: the maternal genome produces maternal proteins that form a gradient in the egg and influence the initial development of the embryo. The zygote’s genome produces proteins that react to these gradient in two ways:
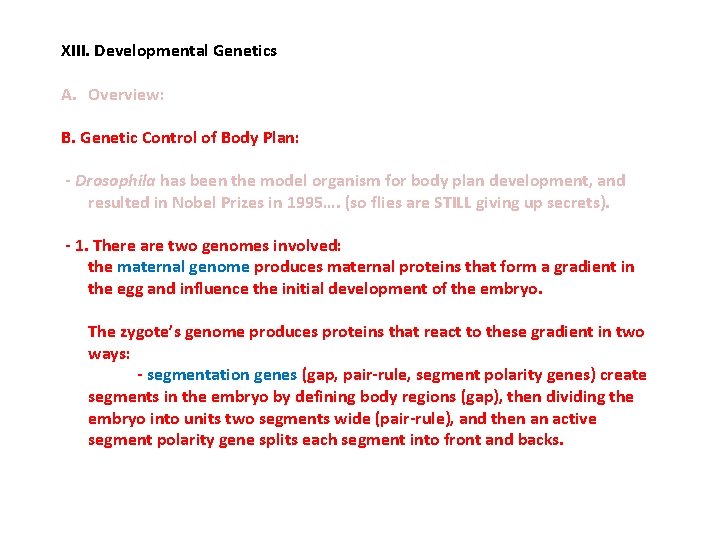
XIII. Developmental Genetics A. Overview: B. Genetic Control of Body Plan: - Drosophila has been the model organism for body plan development, and resulted in Nobel Prizes in 1995…. (so flies are STILL giving up secrets). - 1. There are two genomes involved: the maternal genome produces maternal proteins that form a gradient in the egg and influence the initial development of the embryo. The zygote’s genome produces proteins that react to these gradient in two ways: - segmentation genes (gap, pair-rule, segment polarity genes) create segments in the embryo by defining body regions (gap), then dividing the embryo into units two segments wide (pair-rule), and then an active segment polarity gene splits each segment into front and backs.
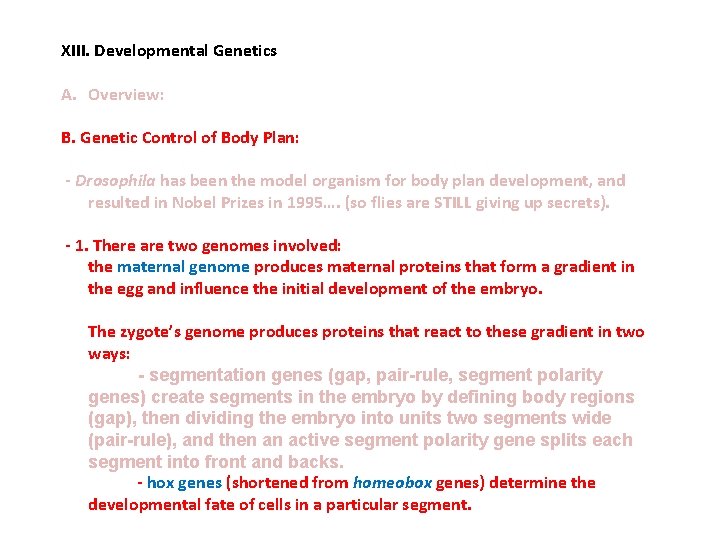
XIII. Developmental Genetics A. Overview: B. Genetic Control of Body Plan: - Drosophila has been the model organism for body plan development, and resulted in Nobel Prizes in 1995…. (so flies are STILL giving up secrets). - 1. There are two genomes involved: the maternal genome produces maternal proteins that form a gradient in the egg and influence the initial development of the embryo. The zygote’s genome produces proteins that react to these gradient in two ways: - segmentation genes (gap, pair-rule, segment polarity genes) create segments in the embryo by defining body regions (gap), then dividing the embryo into units two segments wide (pair-rule), and then an active segment polarity gene splits each segment into front and backs. - hox genes (shortened from homeobox genes) determine the developmental fate of cells in a particular segment.
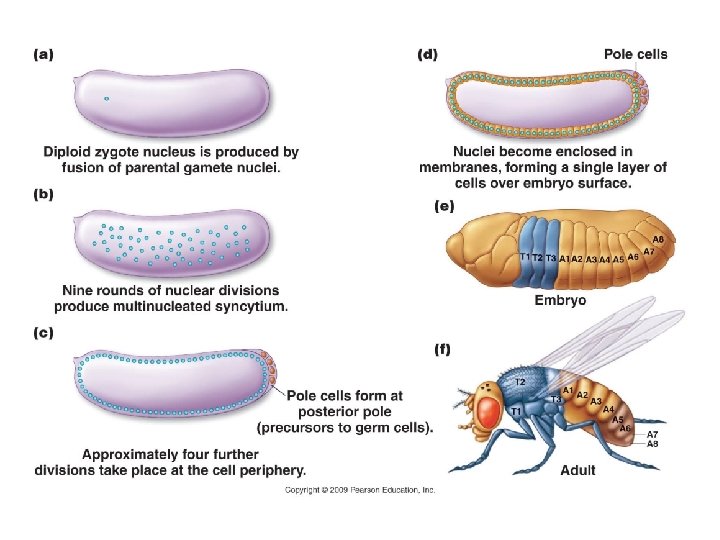
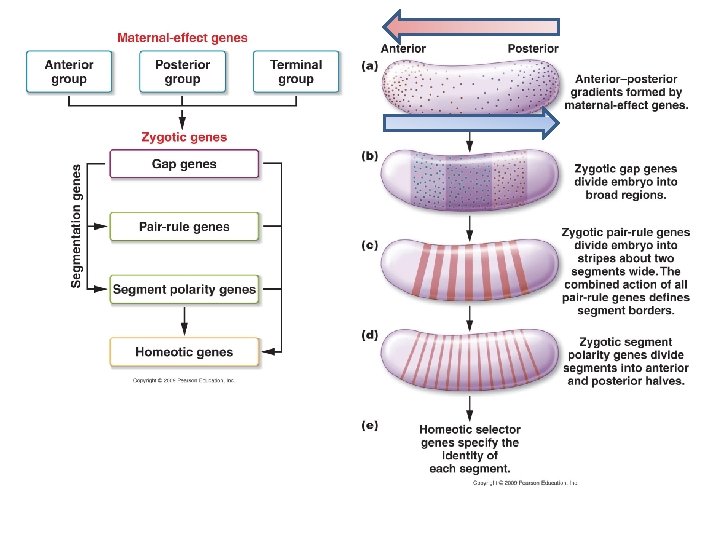
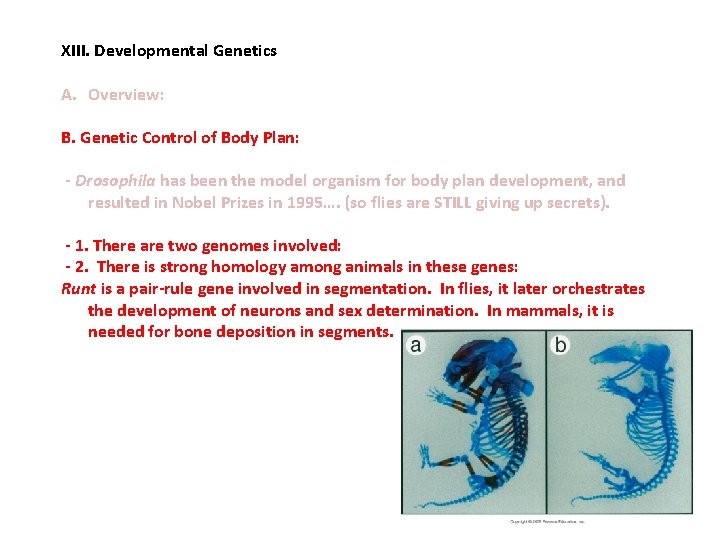
XIII. Developmental Genetics A. Overview: B. Genetic Control of Body Plan: - Drosophila has been the model organism for body plan development, and resulted in Nobel Prizes in 1995…. (so flies are STILL giving up secrets). - 1. There are two genomes involved: - 2. There is strong homology among animals in these genes: Runt is a pair-rule gene involved in segmentation. In flies, it later orchestrates the development of neurons and sex determination. In mammals, it is needed for bone deposition in segments.
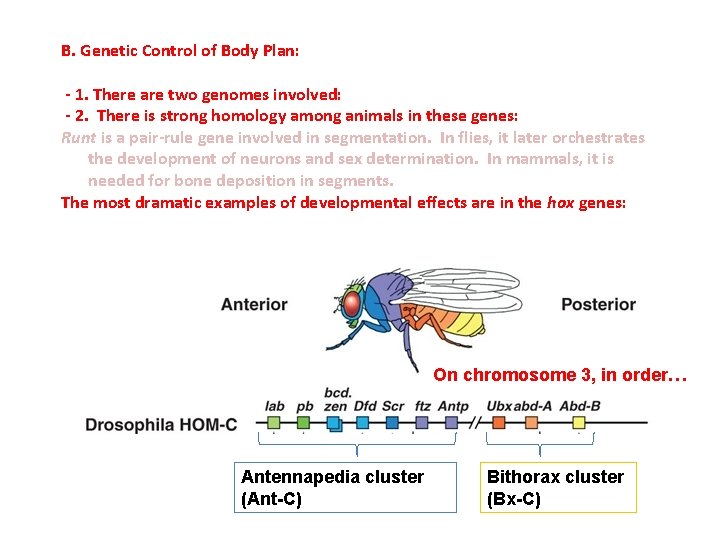
B. Genetic Control of Body Plan: - 1. There are two genomes involved: - 2. There is strong homology among animals in these genes: Runt is a pair-rule gene involved in segmentation. In flies, it later orchestrates the development of neurons and sex determination. In mammals, it is needed for bone deposition in segments. The most dramatic examples of developmental effects are in the hox genes: On chromosome 3, in order… Antennapedia cluster (Ant-C) Bithorax cluster (Bx-C)
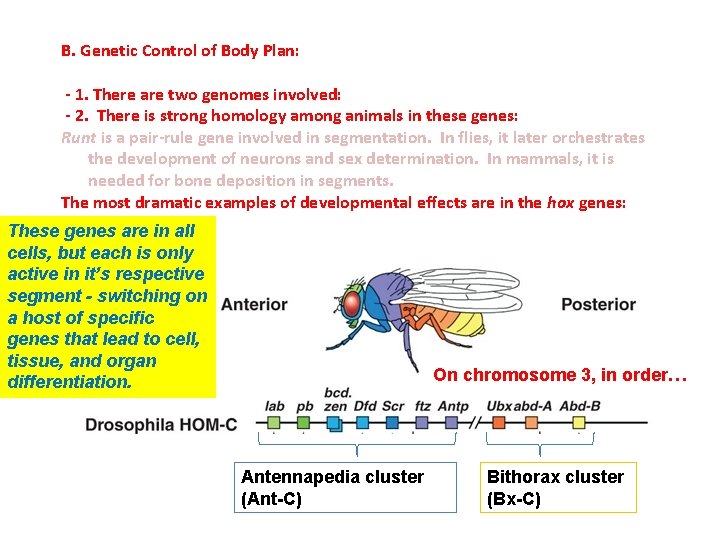
B. Genetic Control of Body Plan: - 1. There are two genomes involved: - 2. There is strong homology among animals in these genes: Runt is a pair-rule gene involved in segmentation. In flies, it later orchestrates the development of neurons and sex determination. In mammals, it is needed for bone deposition in segments. The most dramatic examples of developmental effects are in the hox genes: These genes are in all cells, but each is only active in it’s respective segment - switching on a host of specific genes that lead to cell, tissue, and organ differentiation. On chromosome 3, in order… Antennapedia cluster (Ant-C) Bithorax cluster (Bx-C)
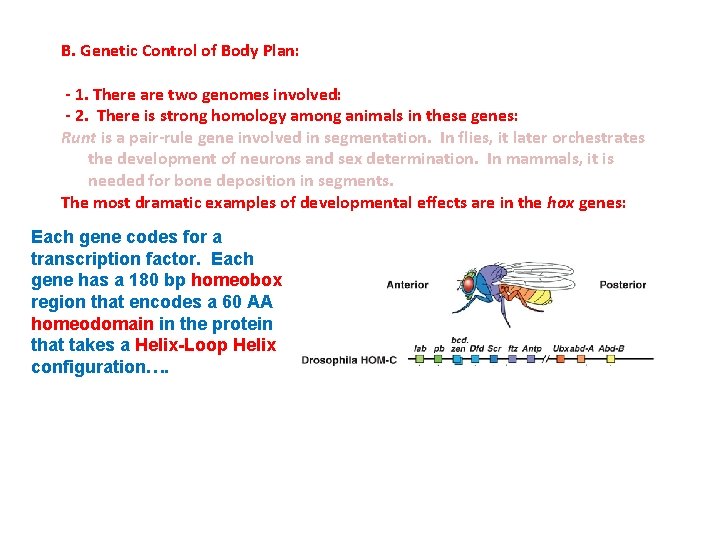
B. Genetic Control of Body Plan: - 1. There are two genomes involved: - 2. There is strong homology among animals in these genes: Runt is a pair-rule gene involved in segmentation. In flies, it later orchestrates the development of neurons and sex determination. In mammals, it is needed for bone deposition in segments. The most dramatic examples of developmental effects are in the hox genes: Each gene codes for a transcription factor. Each gene has a 180 bp homeobox region that encodes a 60 AA homeodomain in the protein that takes a Helix-Loop Helix configuration….
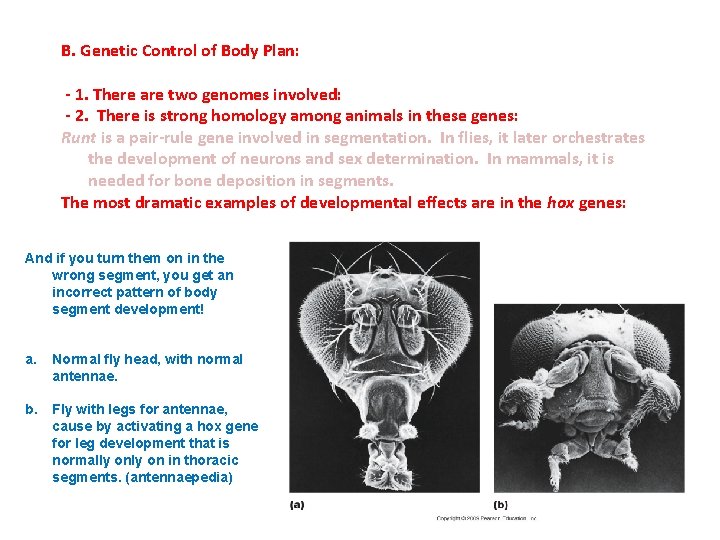
B. Genetic Control of Body Plan: - 1. There are two genomes involved: - 2. There is strong homology among animals in these genes: Runt is a pair-rule gene involved in segmentation. In flies, it later orchestrates the development of neurons and sex determination. In mammals, it is needed for bone deposition in segments. The most dramatic examples of developmental effects are in the hox genes: And if you turn them on in the wrong segment, you get an incorrect pattern of body segment development! a. Normal fly head, with normal antennae. b. Fly with legs for antennae, cause by activating a hox gene for leg development that is normally on in thoracic segments. (antennaepedia)
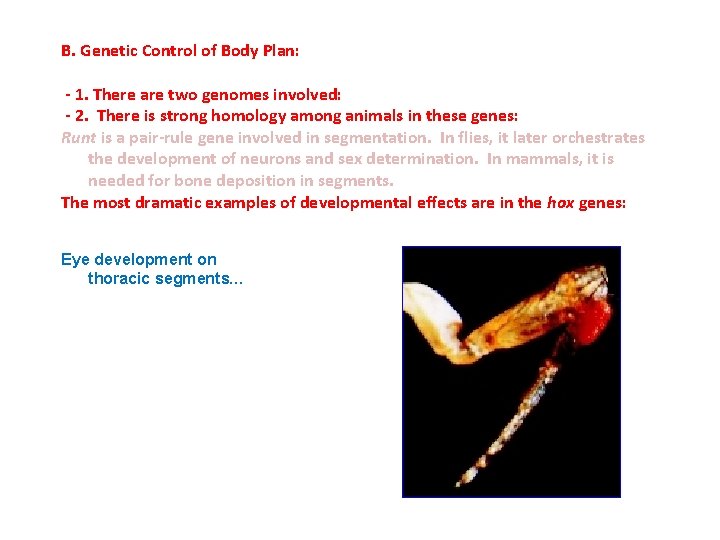
B. Genetic Control of Body Plan: - 1. There are two genomes involved: - 2. There is strong homology among animals in these genes: Runt is a pair-rule gene involved in segmentation. In flies, it later orchestrates the development of neurons and sex determination. In mammals, it is needed for bone deposition in segments. The most dramatic examples of developmental effects are in the hox genes: Eye development on thoracic segments…
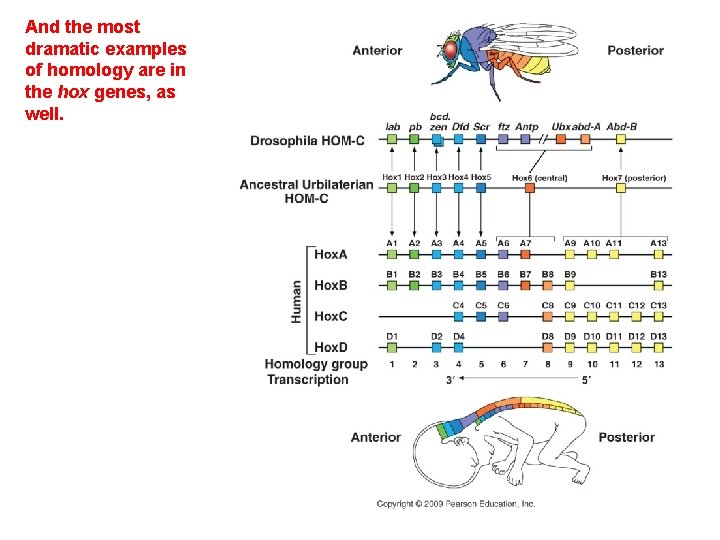
And the most dramatic examples of homology are in the hox genes, as well.
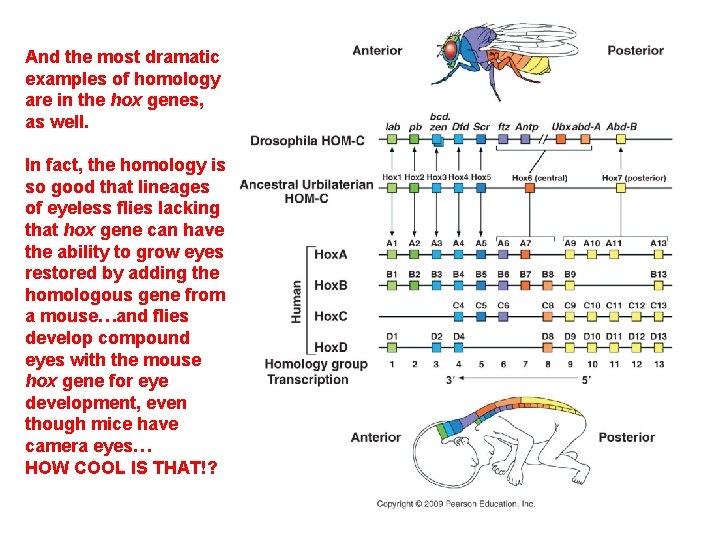
And the most dramatic examples of homology are in the hox genes, as well. In fact, the homology is so good that lineages of eyeless flies lacking that hox gene can have the ability to grow eyes restored by adding the homologous gene from a mouse…and flies develop compound eyes with the mouse hox gene for eye development, even though mice have camera eyes… HOW COOL IS THAT!?
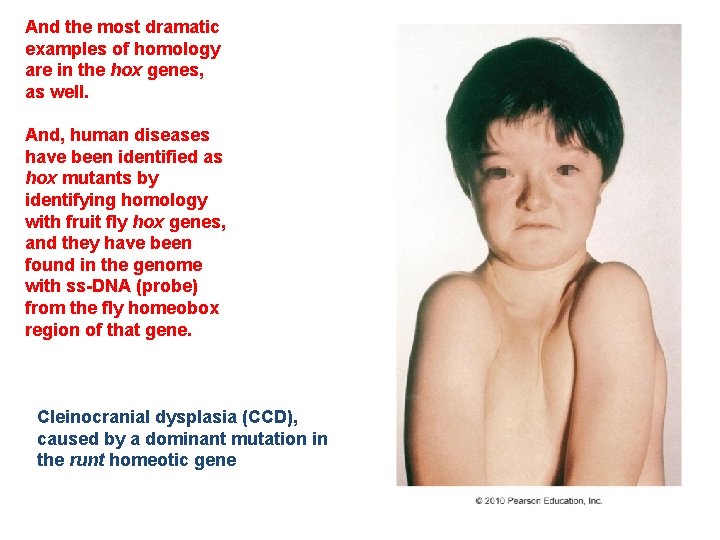
And the most dramatic examples of homology are in the hox genes, as well. And, human diseases have been identified as hox mutants by identifying homology with fruit fly hox genes, and they have been found in the genome with ss-DNA (probe) from the fly homeobox region of that gene. Cleinocranial dysplasia (CCD), caused by a dominant mutation in the runt homeotic gene
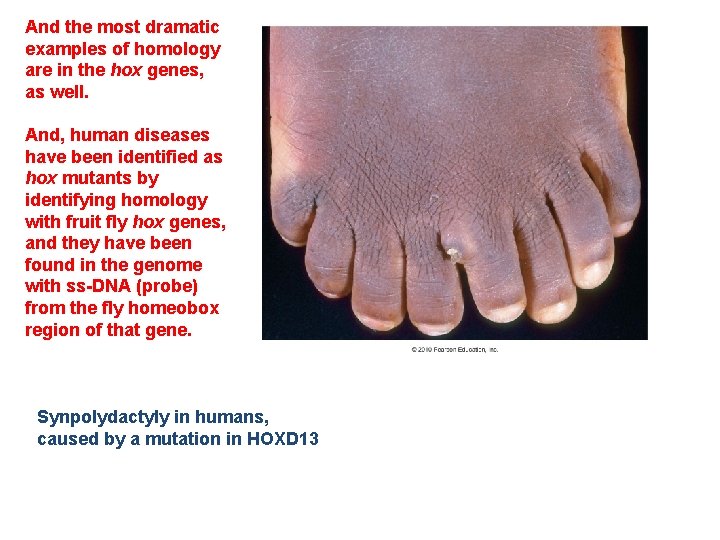
And the most dramatic examples of homology are in the hox genes, as well. And, human diseases have been identified as hox mutants by identifying homology with fruit fly hox genes, and they have been found in the genome with ss-DNA (probe) from the fly homeobox region of that gene. Synpolydactyly in humans, caused by a mutation in HOXD 13
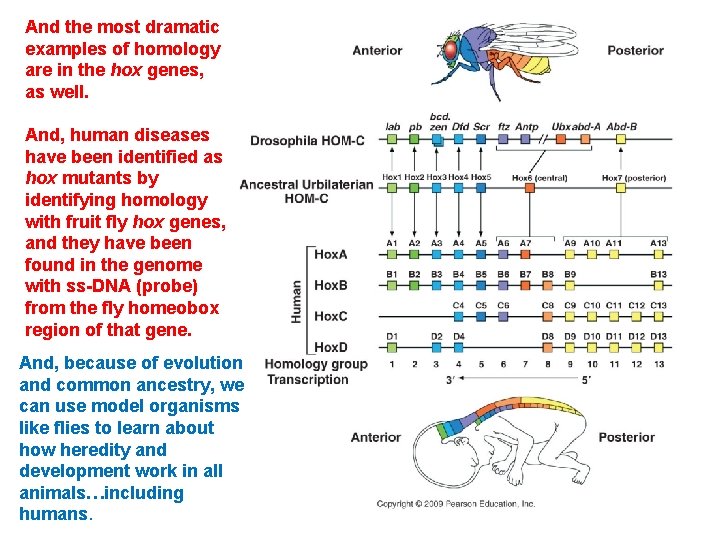
And the most dramatic examples of homology are in the hox genes, as well. And, human diseases have been identified as hox mutants by identifying homology with fruit fly hox genes, and they have been found in the genome with ss-DNA (probe) from the fly homeobox region of that gene. And, because of evolution and common ancestry, we can use model organisms like flies to learn about how heredity and development work in all animals…including humans.
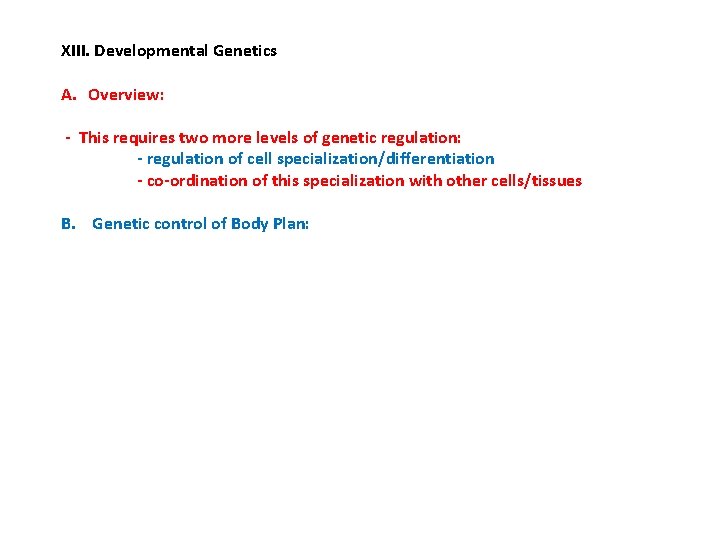
XIII. Developmental Genetics A. Overview: - This requires two more levels of genetic regulation: - regulation of cell specialization/differentiation - co-ordination of this specialization with other cells/tissues B. Genetic control of Body Plan:
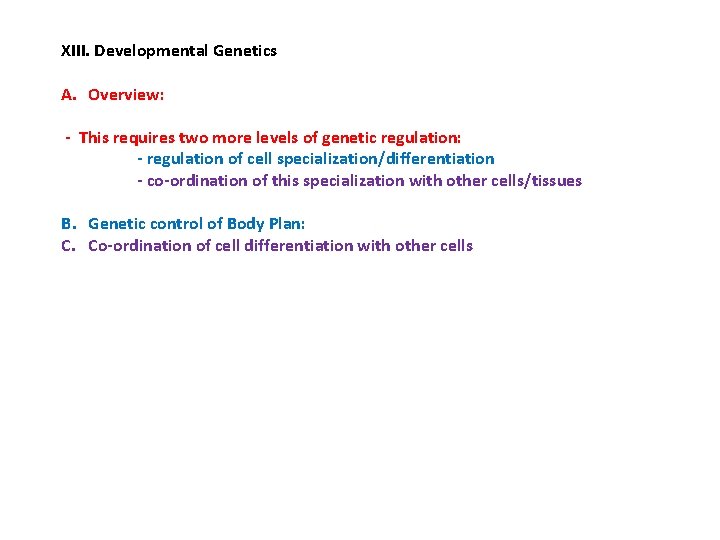
XIII. Developmental Genetics A. Overview: - This requires two more levels of genetic regulation: - regulation of cell specialization/differentiation - co-ordination of this specialization with other cells/tissues B. Genetic control of Body Plan: C. Co-ordination of cell differentiation with other cells
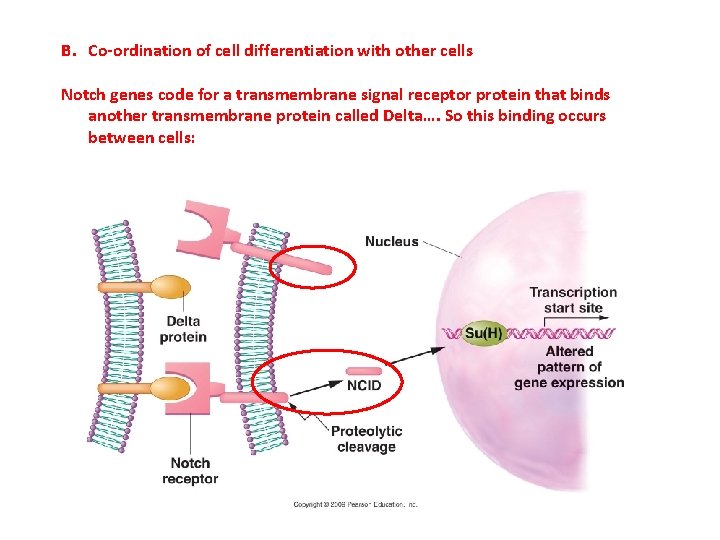
B. Co-ordination of cell differentiation with other cells Notch genes code for a transmembrane signal receptor protein that binds another transmembrane protein called Delta…. So this binding occurs between cells:
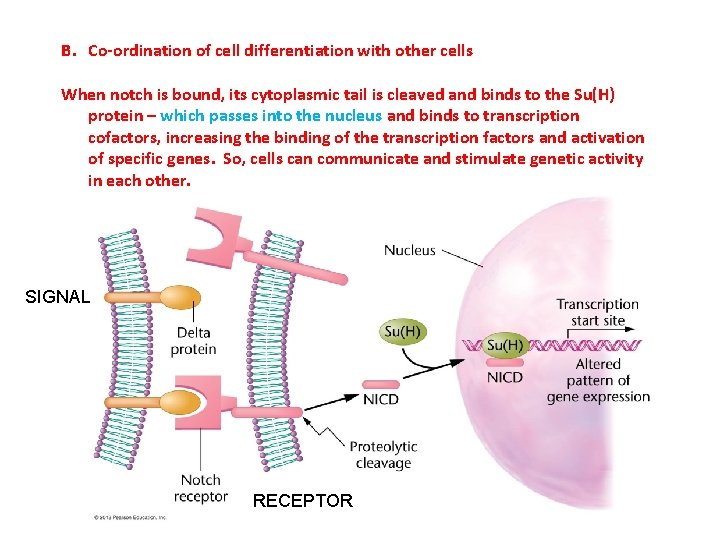
B. Co-ordination of cell differentiation with other cells When notch is bound, its cytoplasmic tail is cleaved and binds to the Su(H) protein – which passes into the nucleus and binds to transcription cofactors, increasing the binding of the transcription factors and activation of specific genes. So, cells can communicate and stimulate genetic activity in each other. SIGNAL RECEPTOR
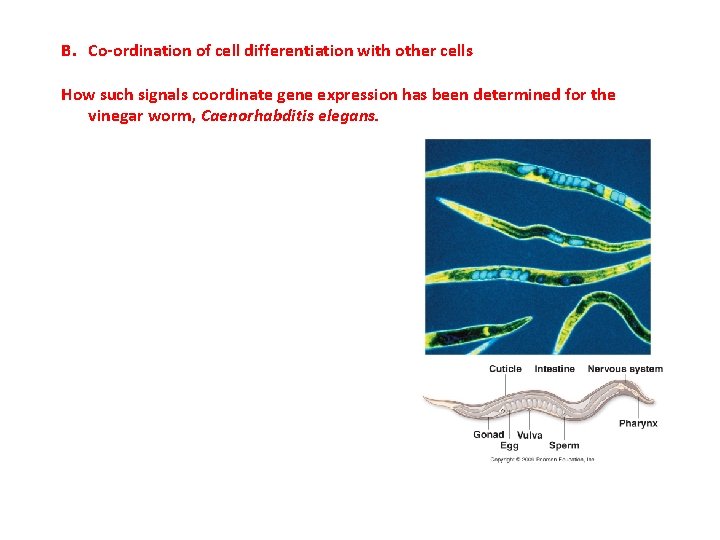
B. Co-ordination of cell differentiation with other cells How such signals coordinate gene expression has been determined for the vinegar worm, Caenorhabditis elegans.
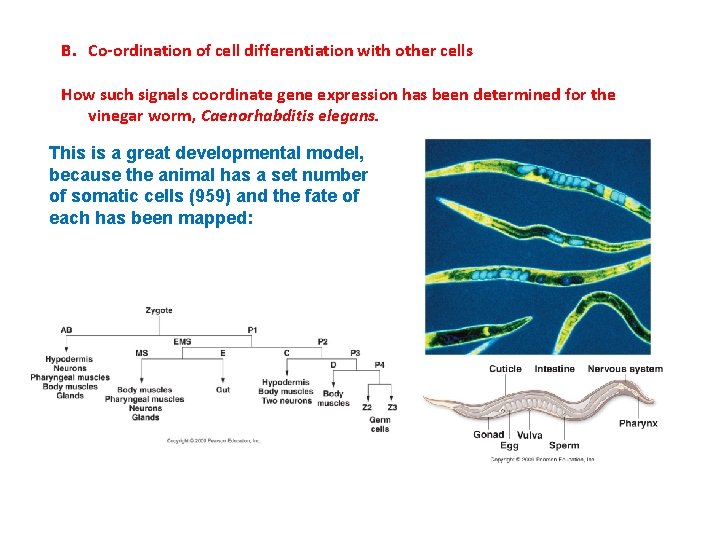
B. Co-ordination of cell differentiation with other cells How such signals coordinate gene expression has been determined for the vinegar worm, Caenorhabditis elegans. This is a great developmental model, because the animal has a set number of somatic cells (959) and the fate of each has been mapped:
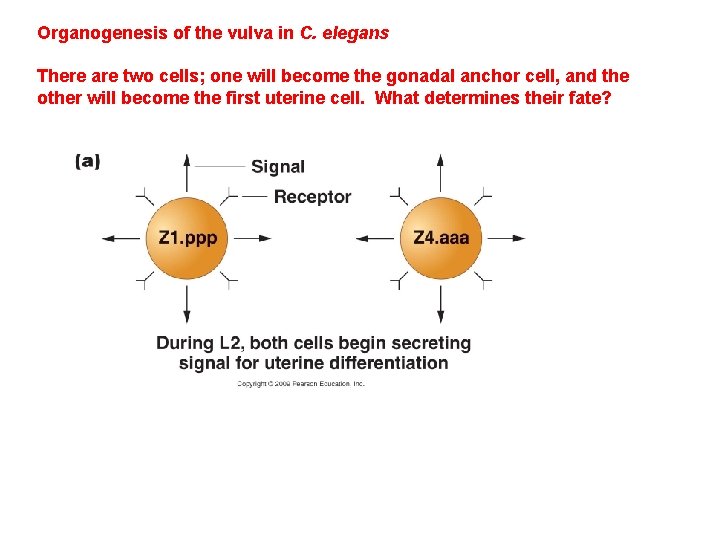
Organogenesis of the vulva in C. elegans There are two cells; one will become the gonadal anchor cell, and the other will become the first uterine cell. What determines their fate?
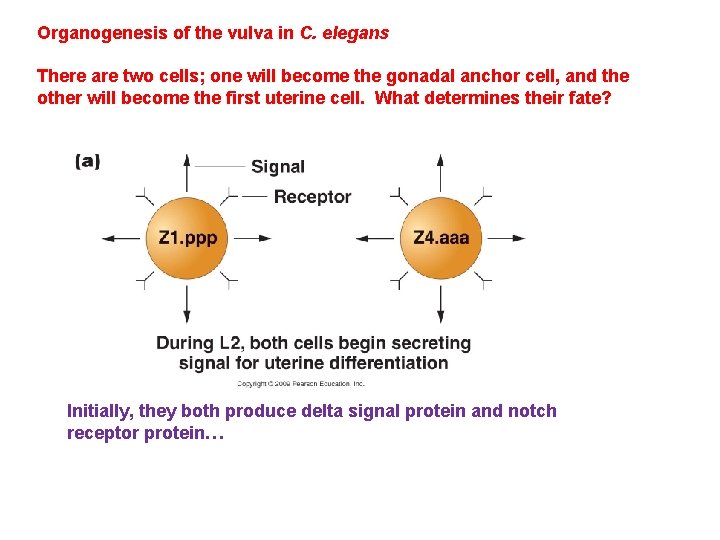
Organogenesis of the vulva in C. elegans There are two cells; one will become the gonadal anchor cell, and the other will become the first uterine cell. What determines their fate? Initially, they both produce delta signal protein and notch receptor protein…
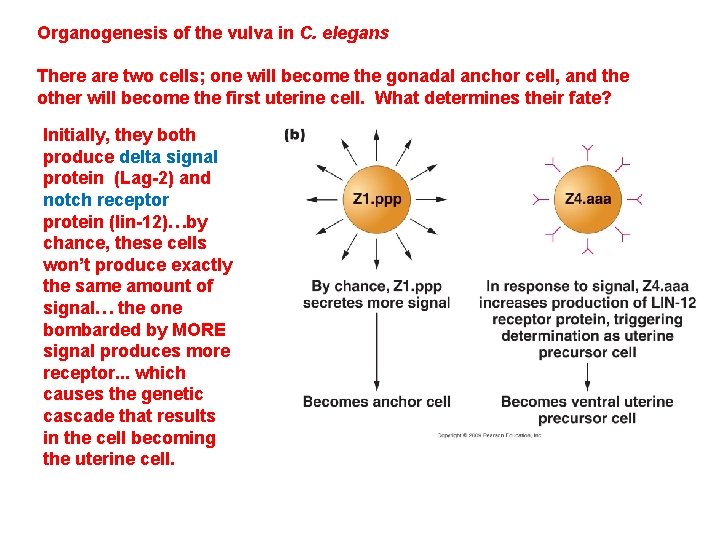
Organogenesis of the vulva in C. elegans There are two cells; one will become the gonadal anchor cell, and the other will become the first uterine cell. What determines their fate? Initially, they both produce delta signal protein (Lag-2) and notch receptor protein (lin-12)…by chance, these cells won’t produce exactly the same amount of signal… the one bombarded by MORE signal produces more receptor. . . which causes the genetic cascade that results in the cell becoming the uterine cell.
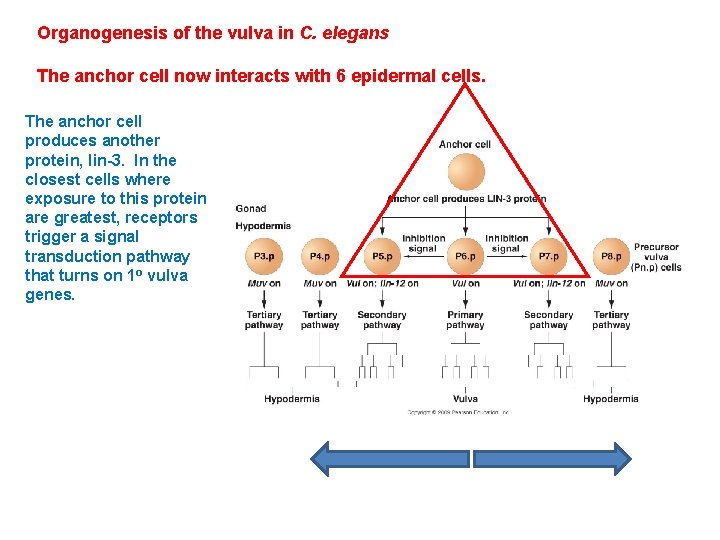
Organogenesis of the vulva in C. elegans The anchor cell now interacts with 6 epidermal cells. The anchor cell produces another protein, lin-3. In the closest cells where exposure to this protein are greatest, receptors trigger a signal transduction pathway that turns on 1 o vulva genes.
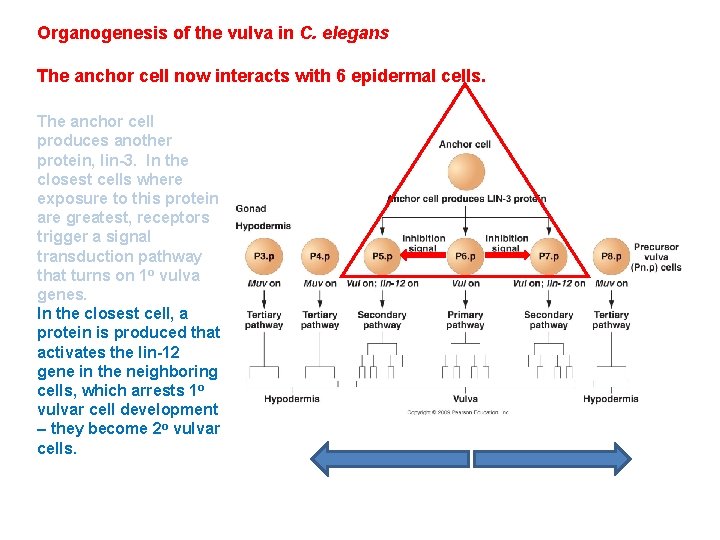
Organogenesis of the vulva in C. elegans The anchor cell now interacts with 6 epidermal cells. The anchor cell produces another protein, lin-3. In the closest cells where exposure to this protein are greatest, receptors trigger a signal transduction pathway that turns on 1 o vulva genes. In the closest cell, a protein is produced that activates the lin-12 gene in the neighboring cells, which arrests 1 o vulvar cell development – they become 2 o vulvar cells.
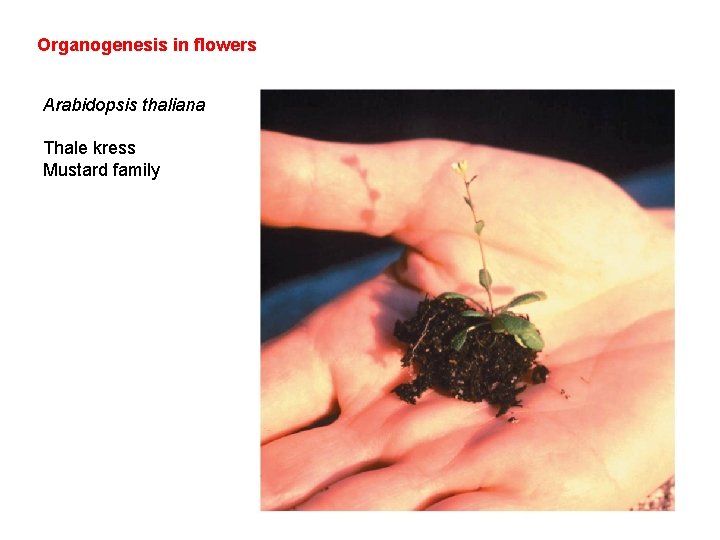
Organogenesis in flowers Arabidopsis thaliana Thale kress Mustard family
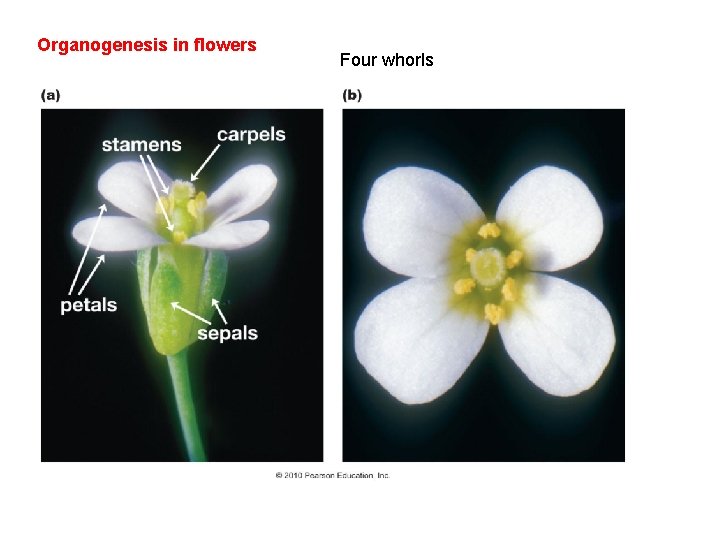
Organogenesis in flowers Four whorls
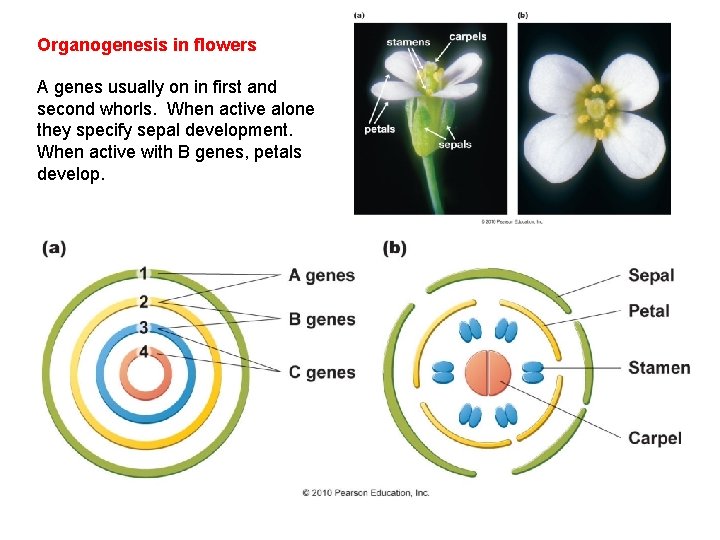
Organogenesis in flowers A genes usually on in first and second whorls. When active alone they specify sepal development. When active with B genes, petals develop.
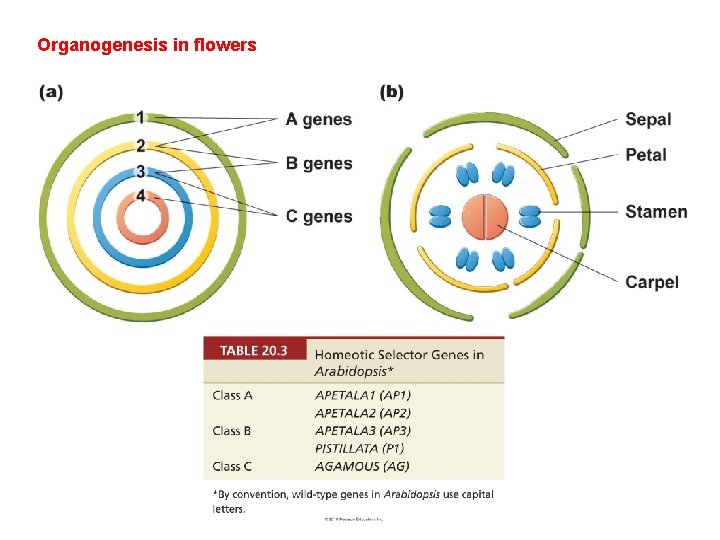
Organogenesis in flowers
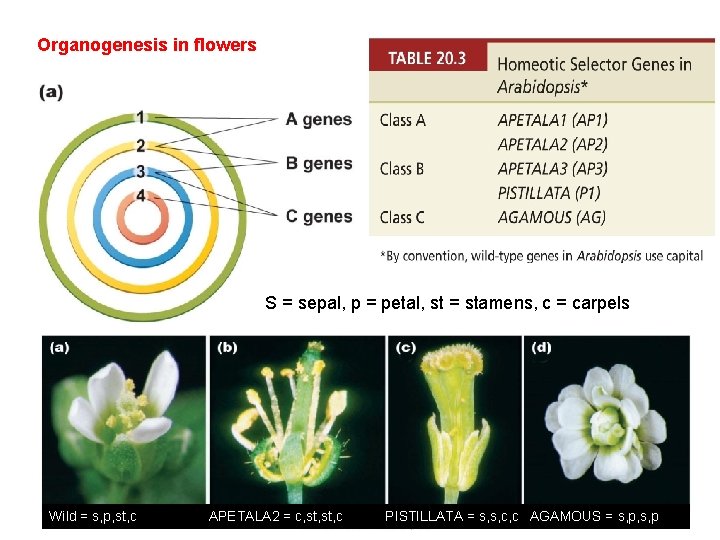
Organogenesis in flowers S = sepal, p = petal, st = stamens, c = carpels Wild = s, p, st, c APETALA 2 = c, st, c PISTILLATA = s, s, c, c AGAMOUS = s, p, s, p
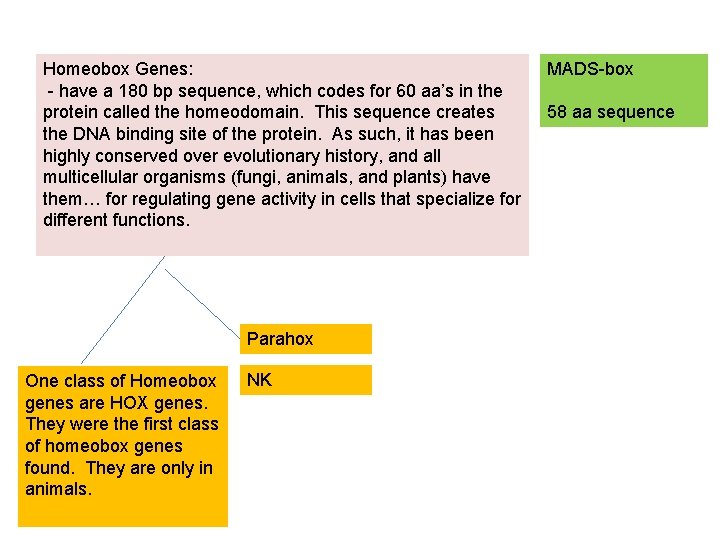
Homeobox Genes: - have a 180 bp sequence, which codes for 60 aa’s in the protein called the homeodomain. This sequence creates the DNA binding site of the protein. As such, it has been highly conserved over evolutionary history, and all multicellular organisms (fungi, animals, and plants) have them… for regulating gene activity in cells that specialize for different functions. Parahox One class of Homeobox genes are HOX genes. They were the first class of homeobox genes found. They are only in animals. NK MADS-box 58 aa sequence
Section 4 gene regulation and mutations
Gene regulation
12-5 gene regulation
Regulation of gene expression in bacteria
Chapter 18 regulation of gene expression
Prokaryotes vs eukaryotes gene regulation
Chapter 18 regulation of gene expression
Chapter 12 section 4: gene regulation and mutations
Regulation of gene expression
Chapter 18 regulation of gene expression
Section 12-5 gene regulation answer key
Chapter 18 regulation of gene expression
What is gene regulation
Gene regulation
Differential gene regulation
Positive control vs negative control operon
Gene by gene test results
"pearson education"
At dna
Olfactory groove keros classification
Reabsorption
Parafollicular cells vs follicular cells
Gametic cells vs somatic cells
Somatic cells vs germ cells
Chlorocruorin
Prokaryotic cells vs eukaryotic cells
Plant cell vs animal cell venn diagram
Prokaryotic cells vs eukaryotic cells
Cell organelle jeopardy
Masses of cells form and steal nutrients from healthy cells
Younger cells cuboidal older cells flattened
What cell type
Are red blood cells prokaryotic
Nondisjunction in meiosis
Cells and life lesson 1 answer key
Name all the rays
Xii lo im. henryka sienkiewicza
Roman numeral twelve
Materi agama katolik kelas xii semester 2
Shemot 11
Viixii
Integral substitusi
Clemens pont max
Legge delle xii tavole
How to test facial nerve function
Francia siglo xii
Stalag allemagne
Sonnet xii
Pope pius xii award
Materi kelas 12 semester 1
Xii lo toruń
Nervus hypogastricus
Col.legi pius xii
Xii tablas
I centauri divina commedia
Ceip pio xii bollullos de la mitacion
Ad altare dei answers chapter 1
Lecturas domingo xii tiempo ordinario ciclo b
Xii domenica del tempo ordinario anno b
Sverige 1658
Nucleus lateralis alae cinereae
Xii number
Ustawa xii tablic
Materi kelas xii semester 1
All cells have
Cell to tissue to organ to organ system to organism
All cells have
All cells must have
All cells exhibit basic structural similarities
What type of cell performs cellular respiration
Help ever hurt never meaning in tamil
Interventi sociali rivolti all'infanzia e all'adolescenza
Above all powers
I work all day i work all night to pay the bills
Communication operations
Sistem all in all out
Spf -all vs ~all
Silent night holy night all is calm
馮定華
All of you is more than enough for all of me
She's all states and all princes i nothing else is
And as he stands in victory sin's curse
Above all powers above all kings
Homometric regulation of cardiac output
Board regulation 1 series of 2014
Line regulation
Jrotc female hair regulations
Countercurrent exchange thermoregulation
Negative feedback and body temperature regulation
Tiffany schuette
Ncoer ar
Army bah regulation 608 99
Table tennis rules and regulations
What is pyloric pump
Regulation of blood pressure
Section 72 of regulation o.reg.941
Prescriptive vs performance
Malaysia elv regulation
Serengeti wildebeest population regulation answer key
Ar 623-3
Jtr, section 0506
Poiseuille's law blood flow
Guided self regulation
What is co regulation
Homeostasis mechanisms for regulation of body temperature
How there is recyclability and self regulation in nature
Model of professional nursing practice regulation
Army mandatory removal date extension
Ncoer regulation ar 623-205
Regulation of blood pressure negative feedback
How to compute mcit