Physics at Hadron Colliders Physics Tevatron Partat III
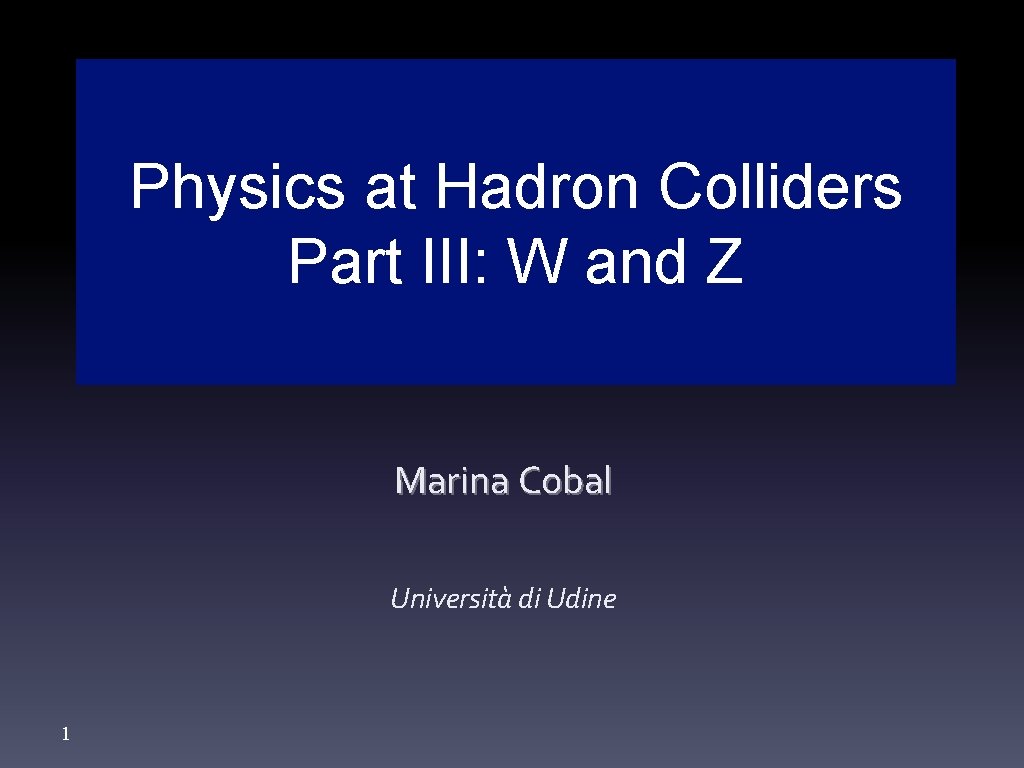
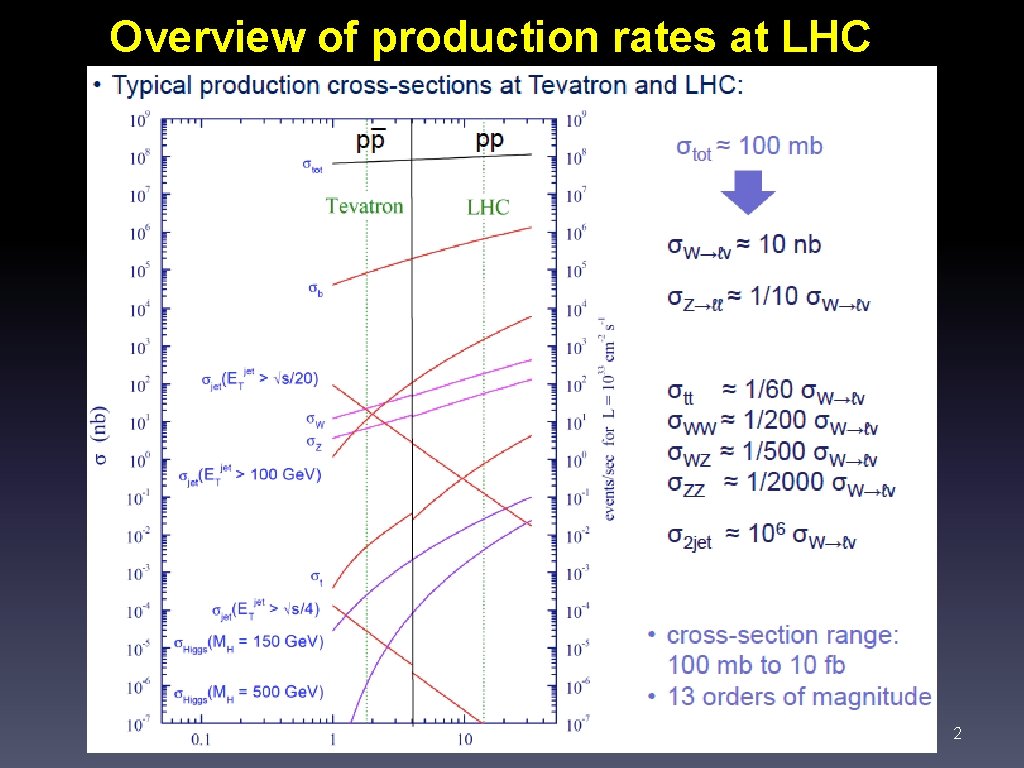
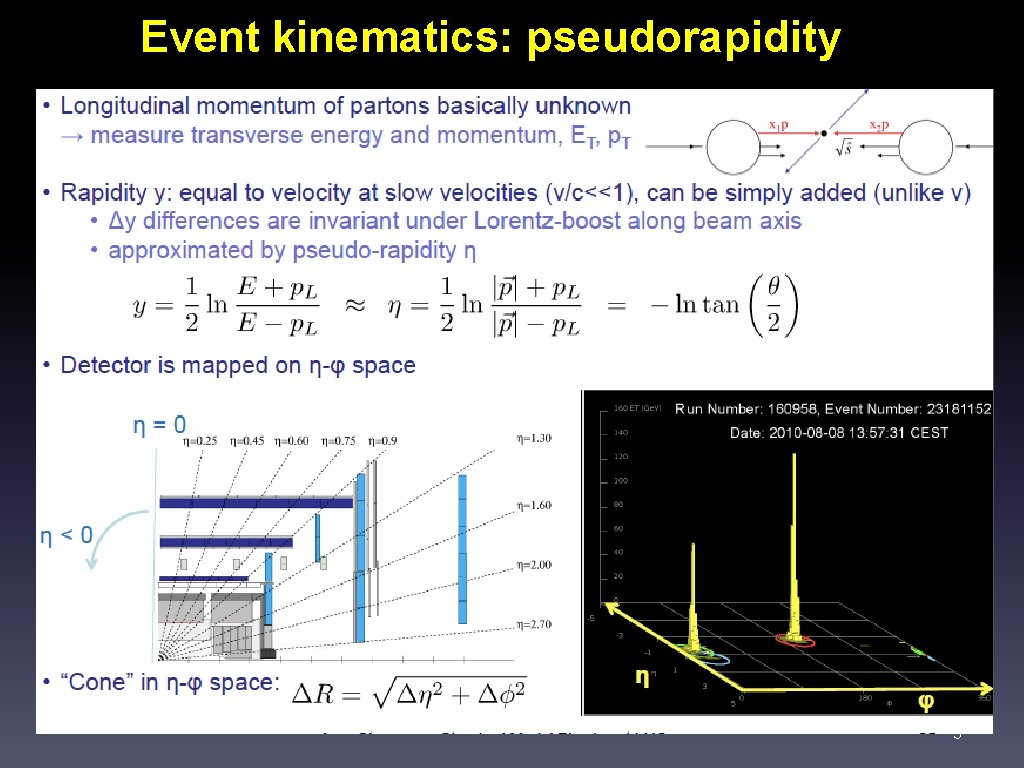
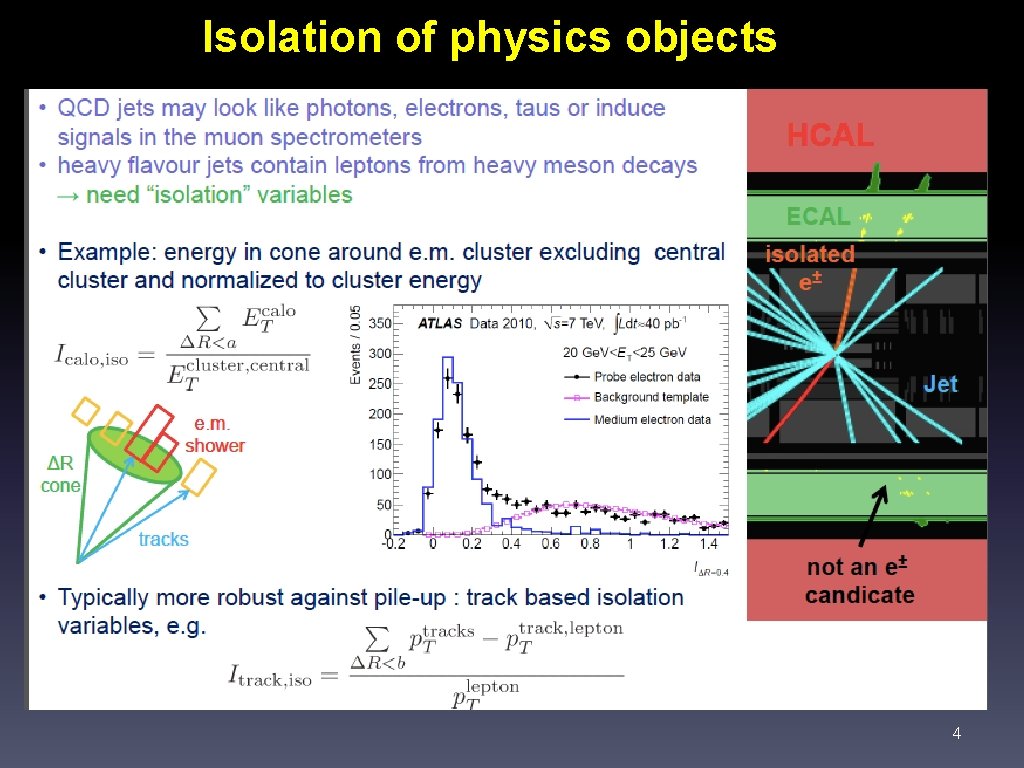
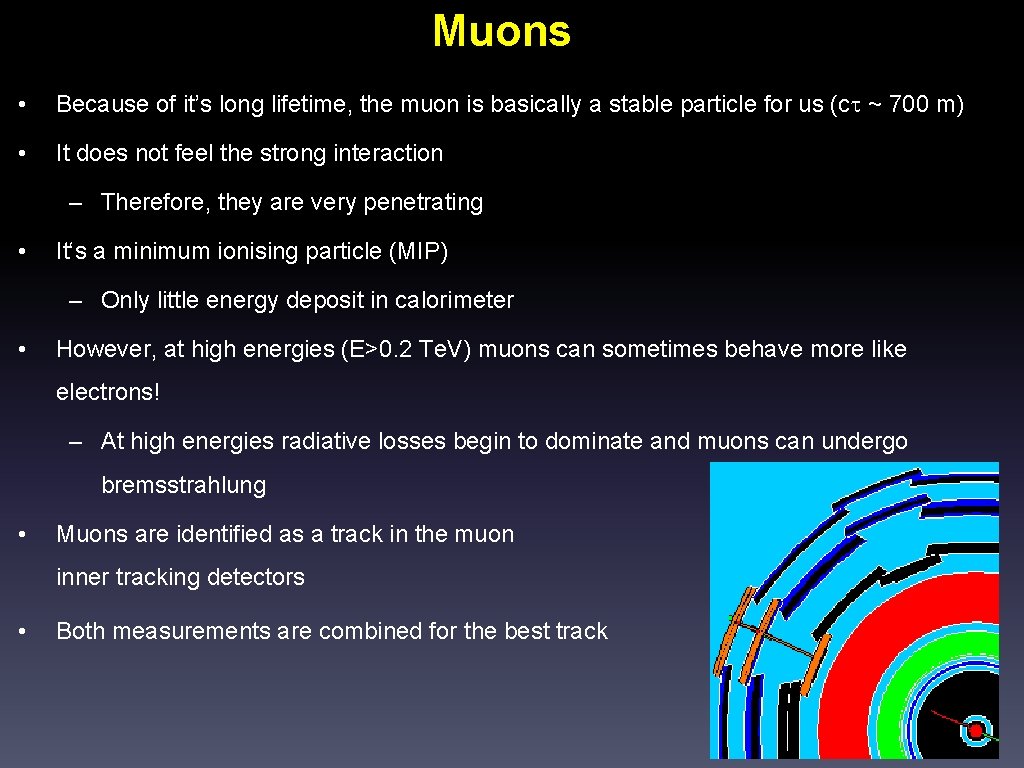
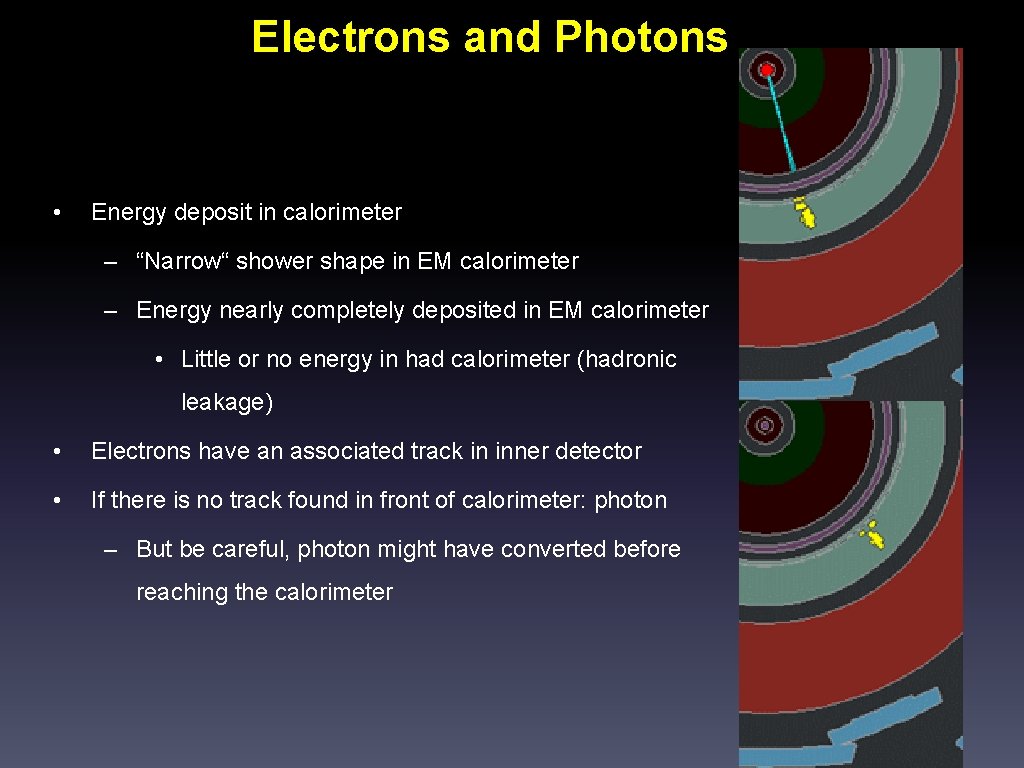
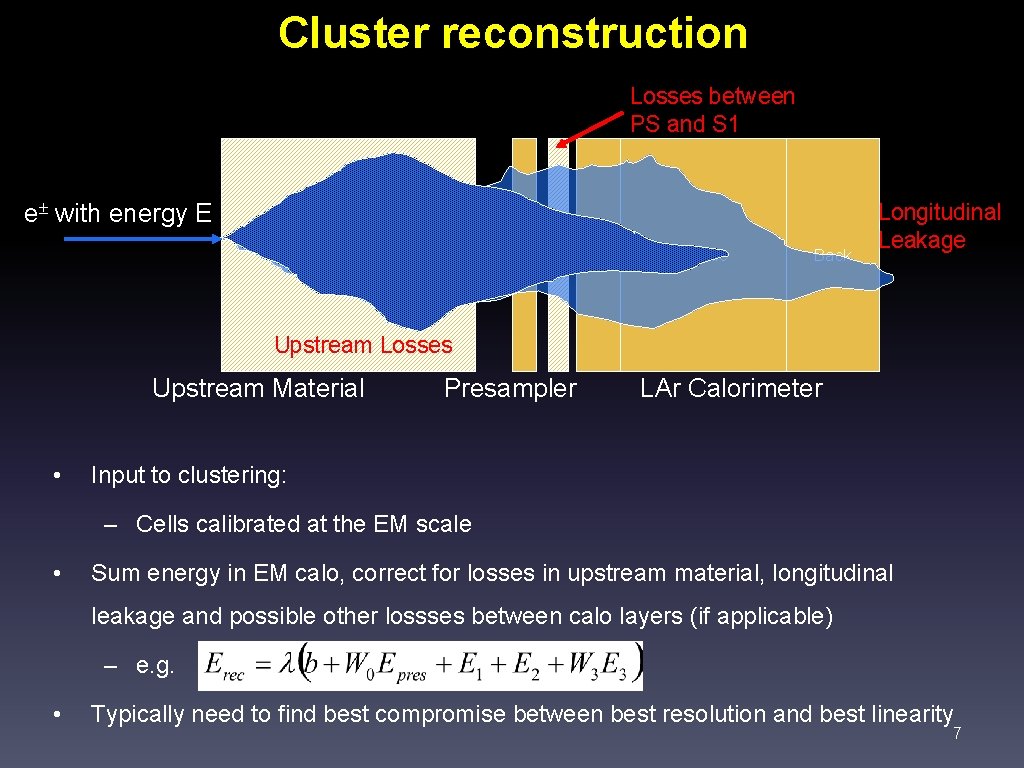
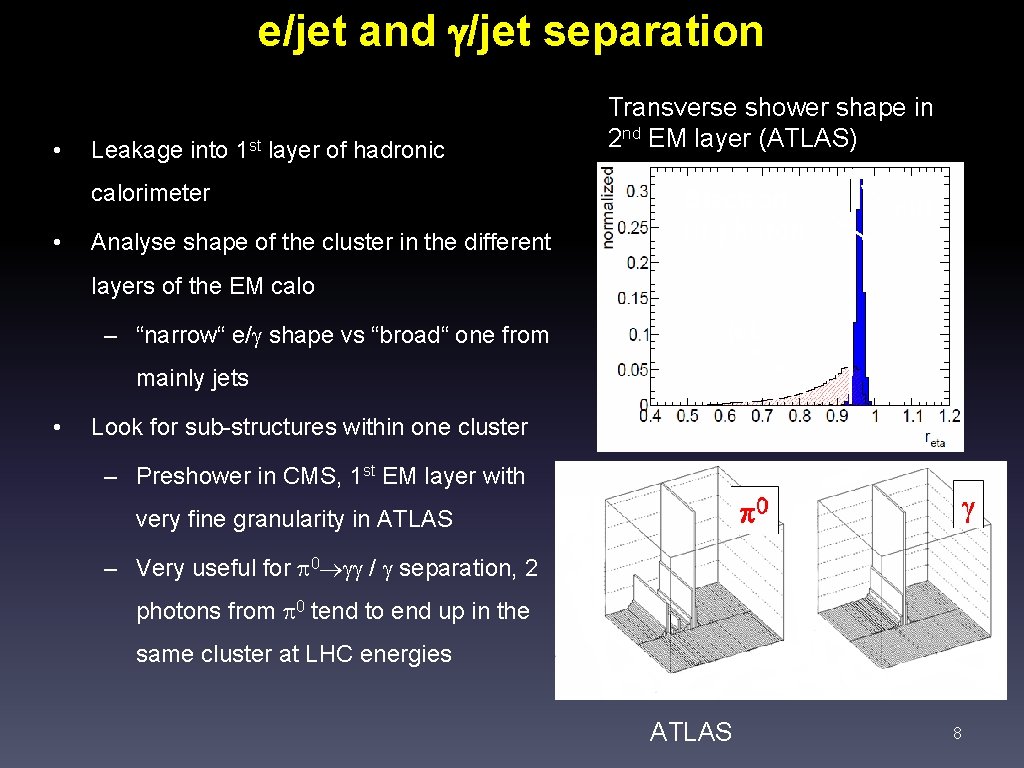
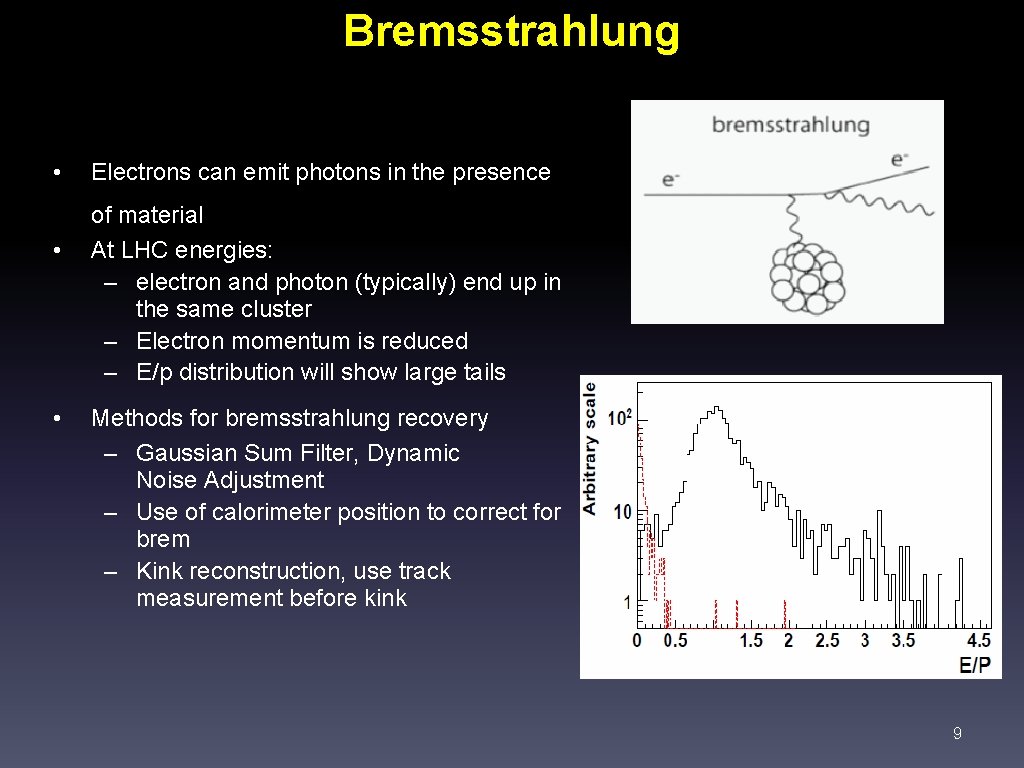
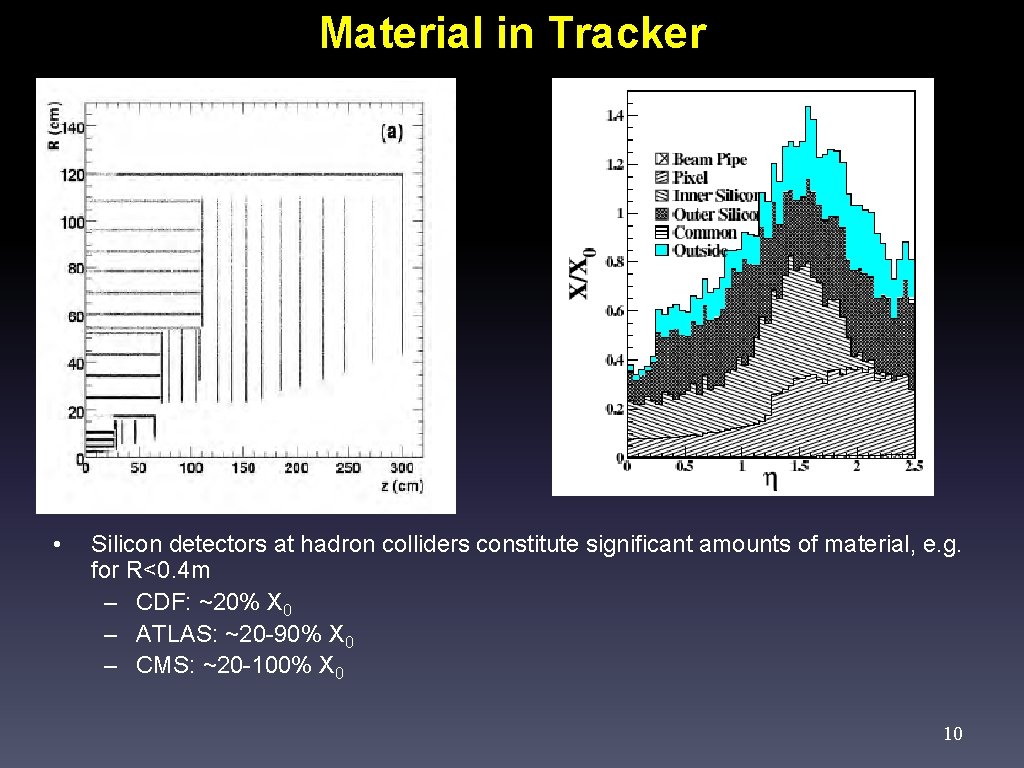
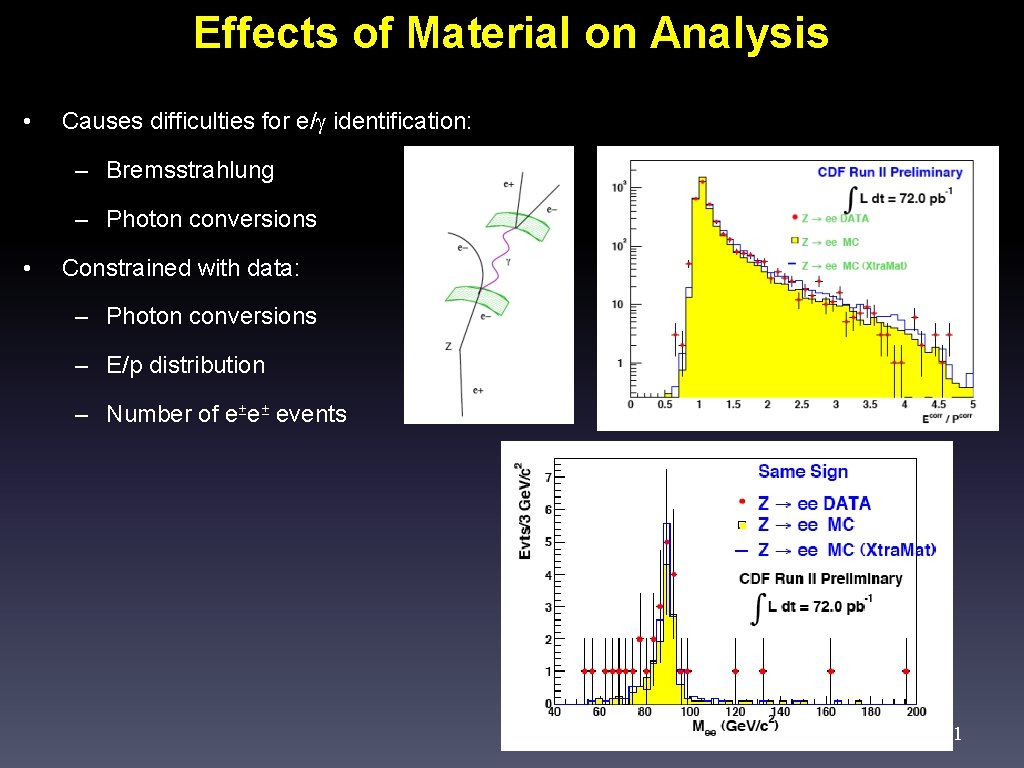
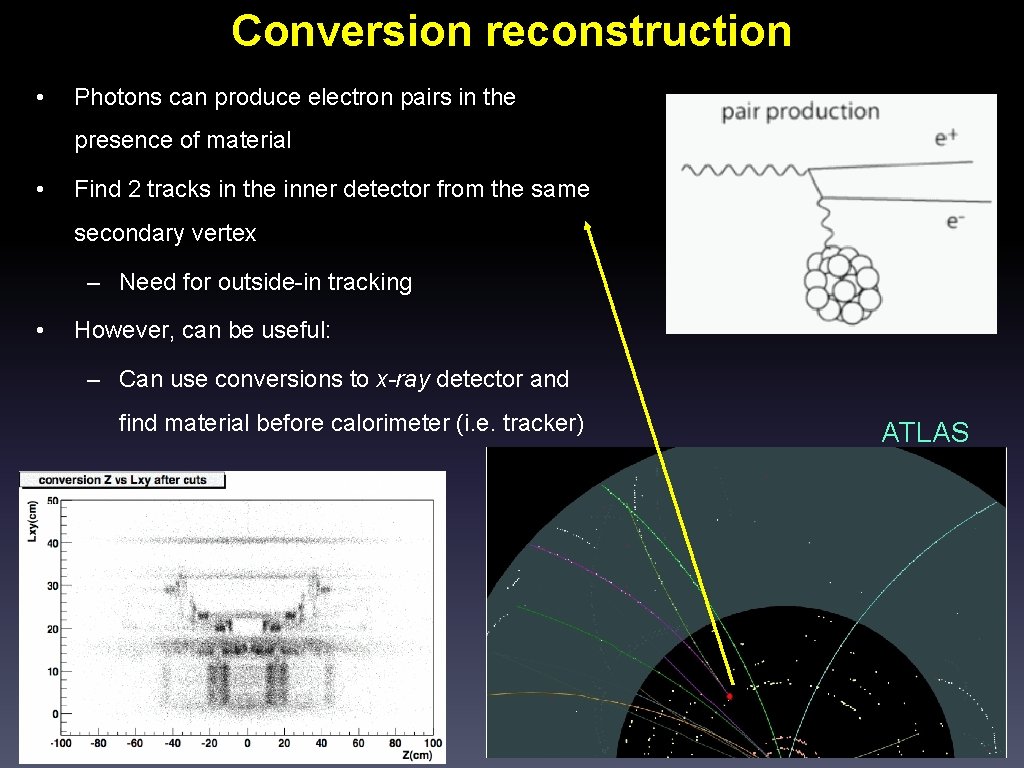
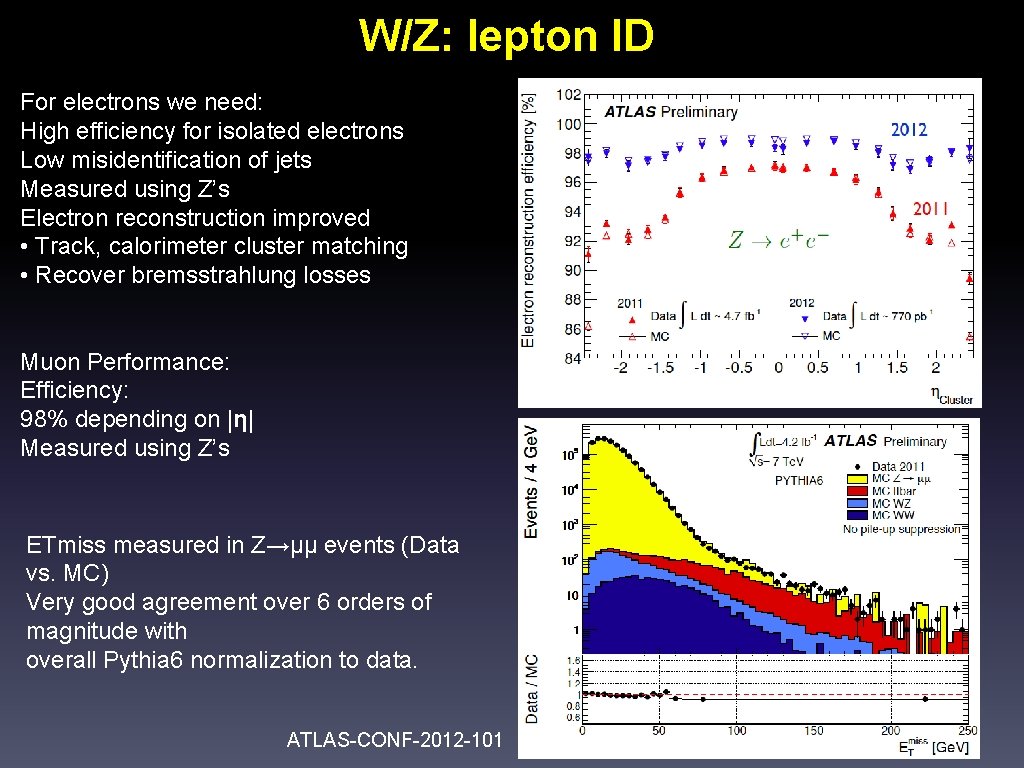
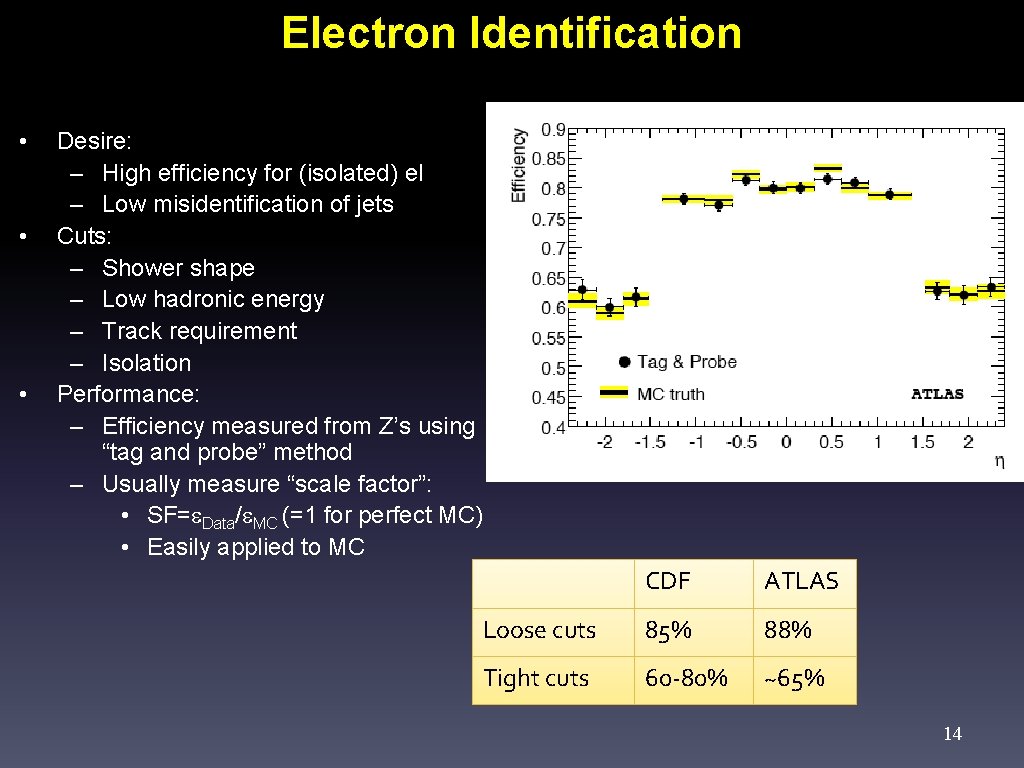
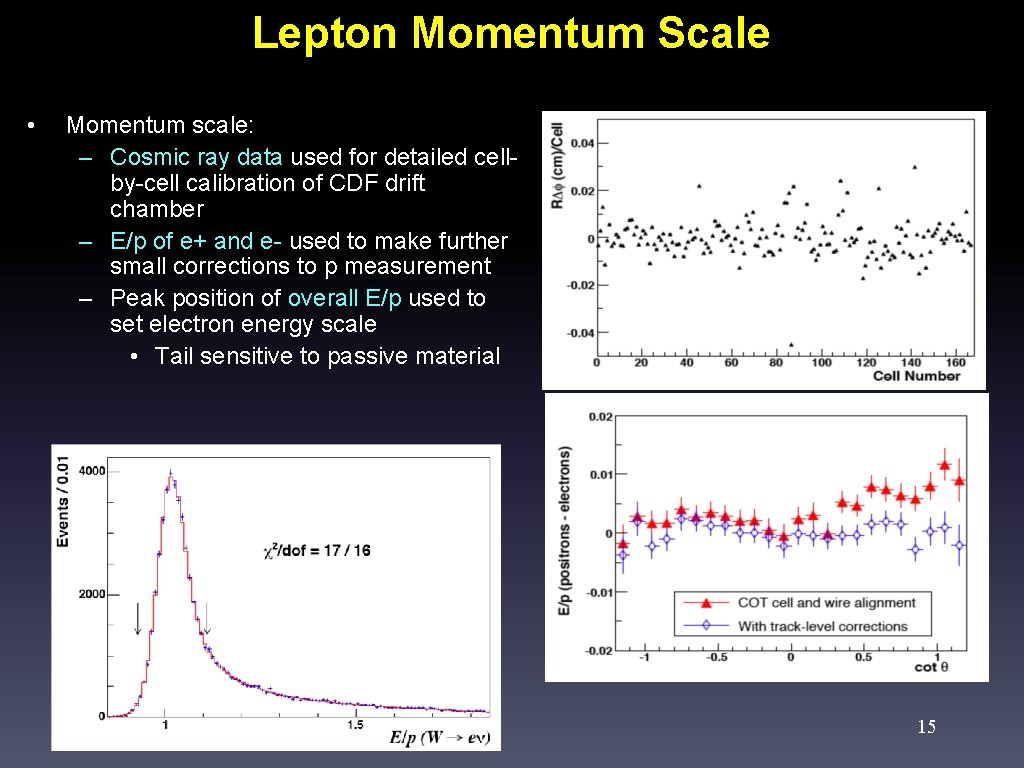
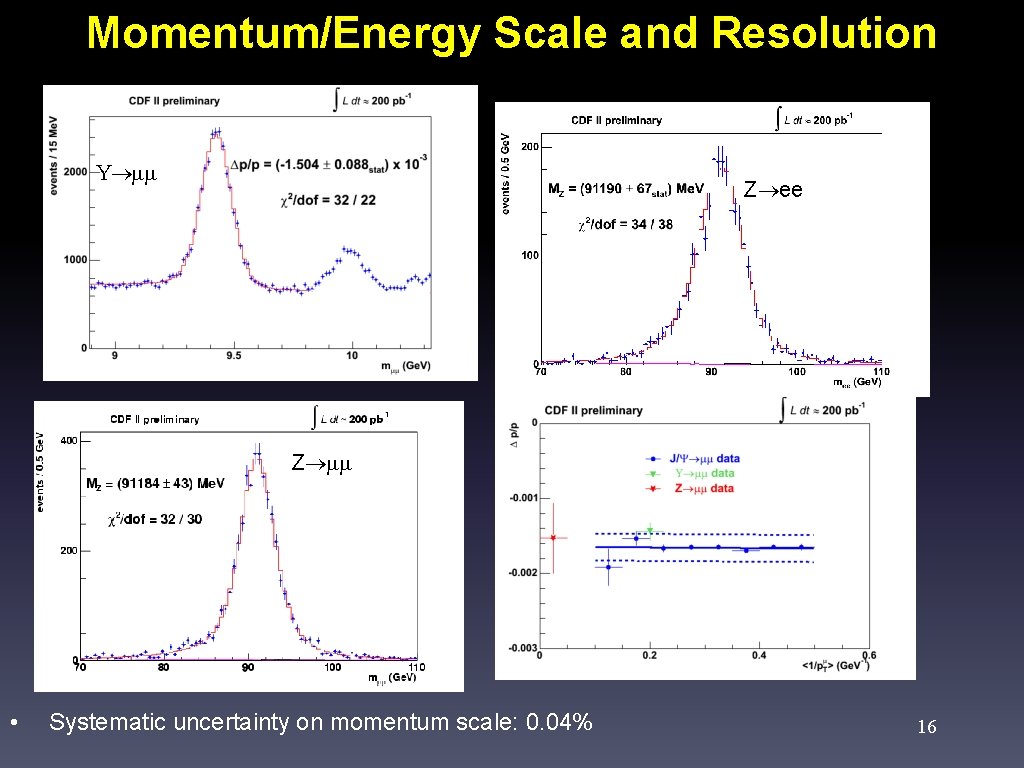
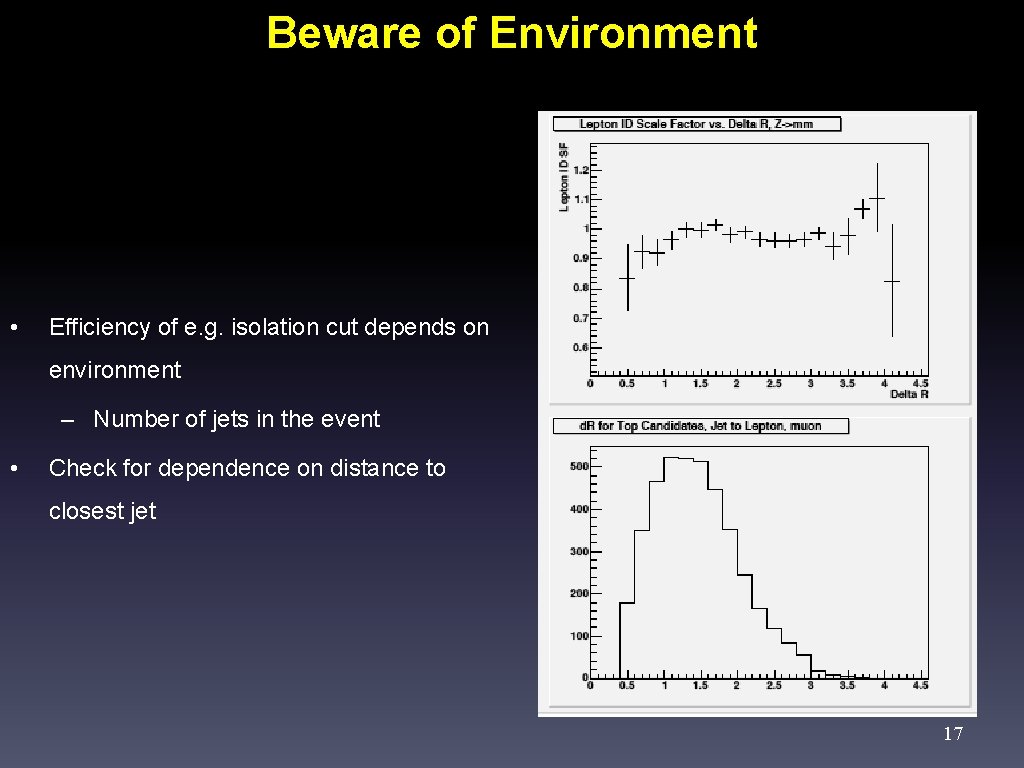
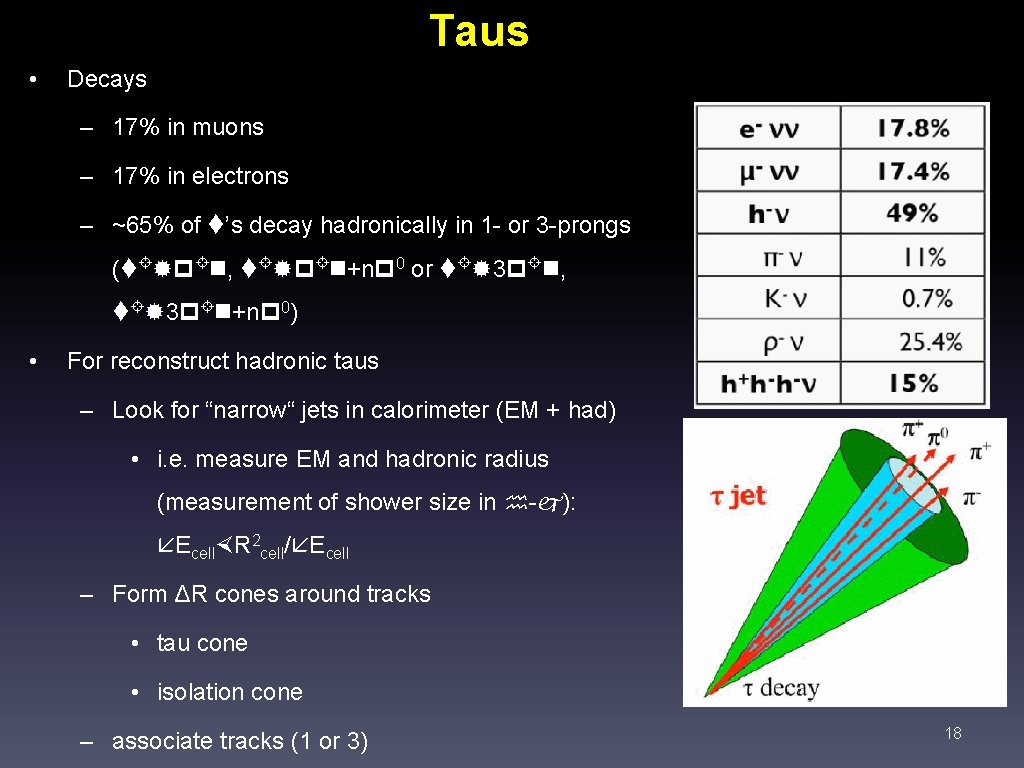
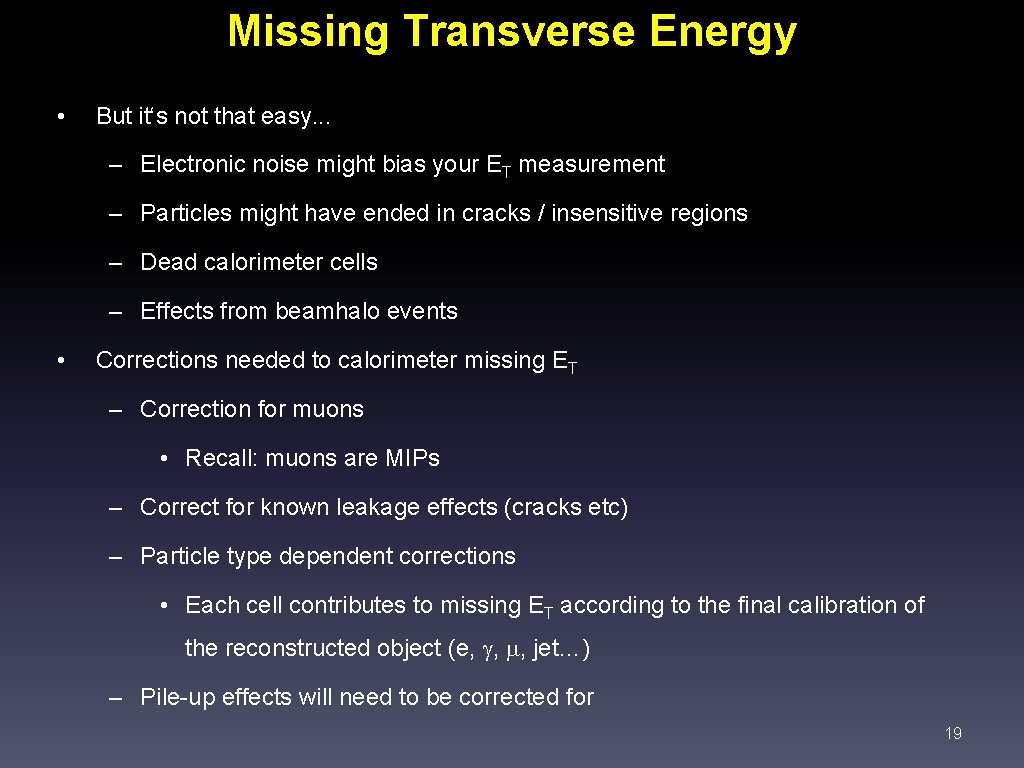
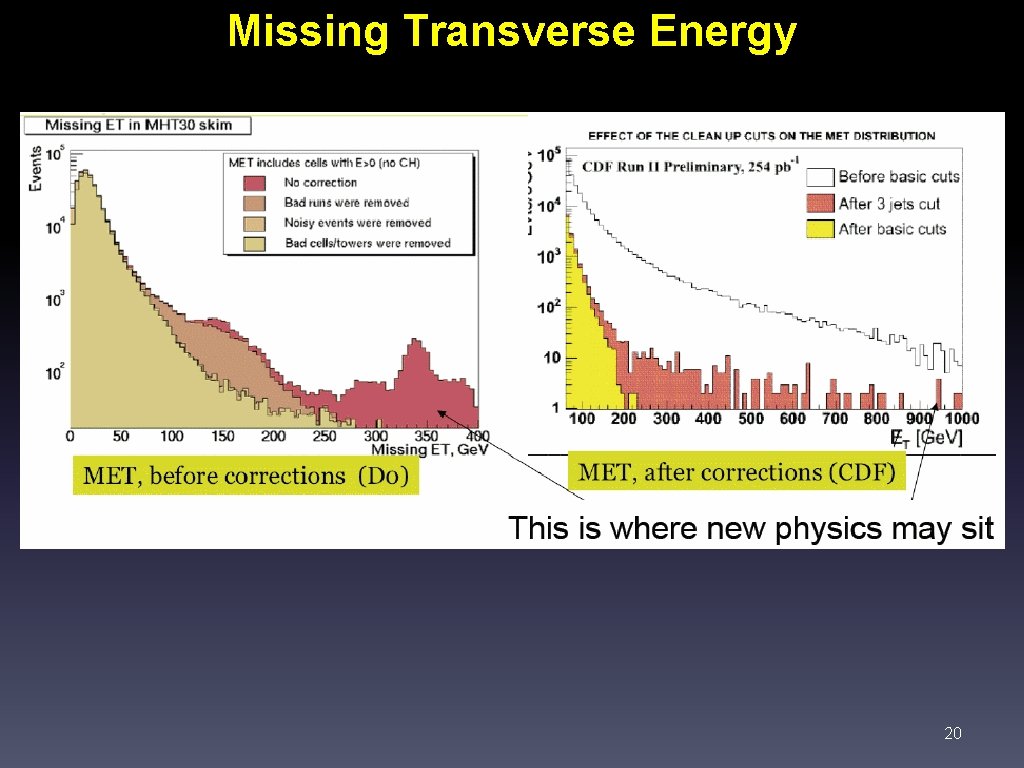
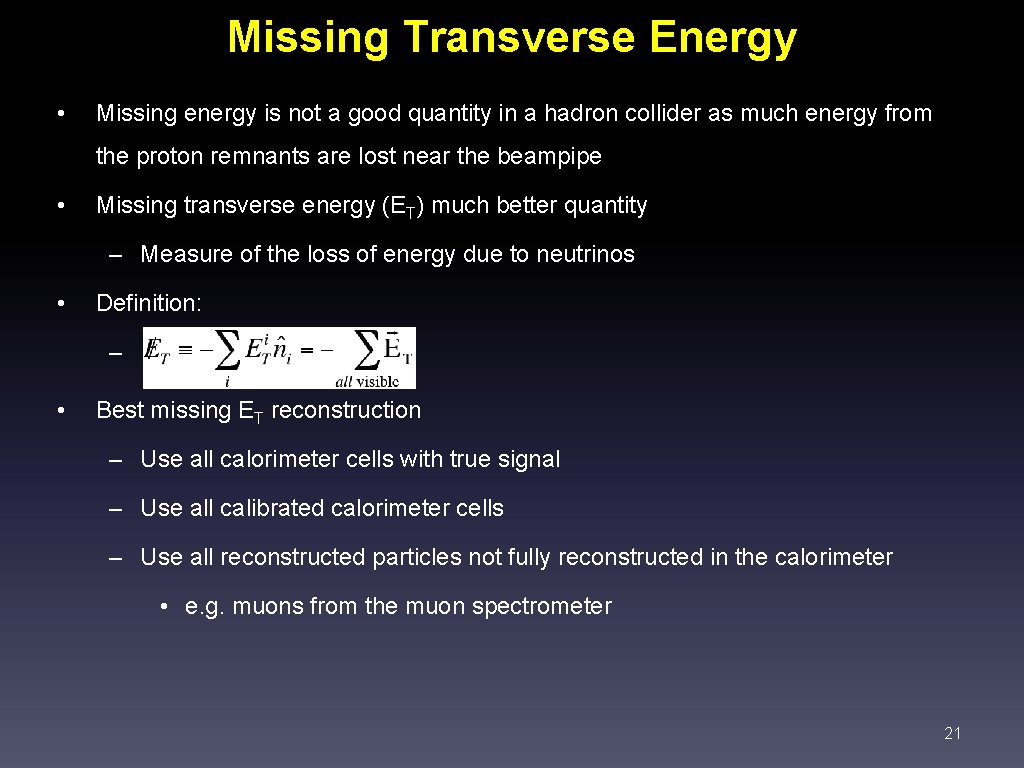
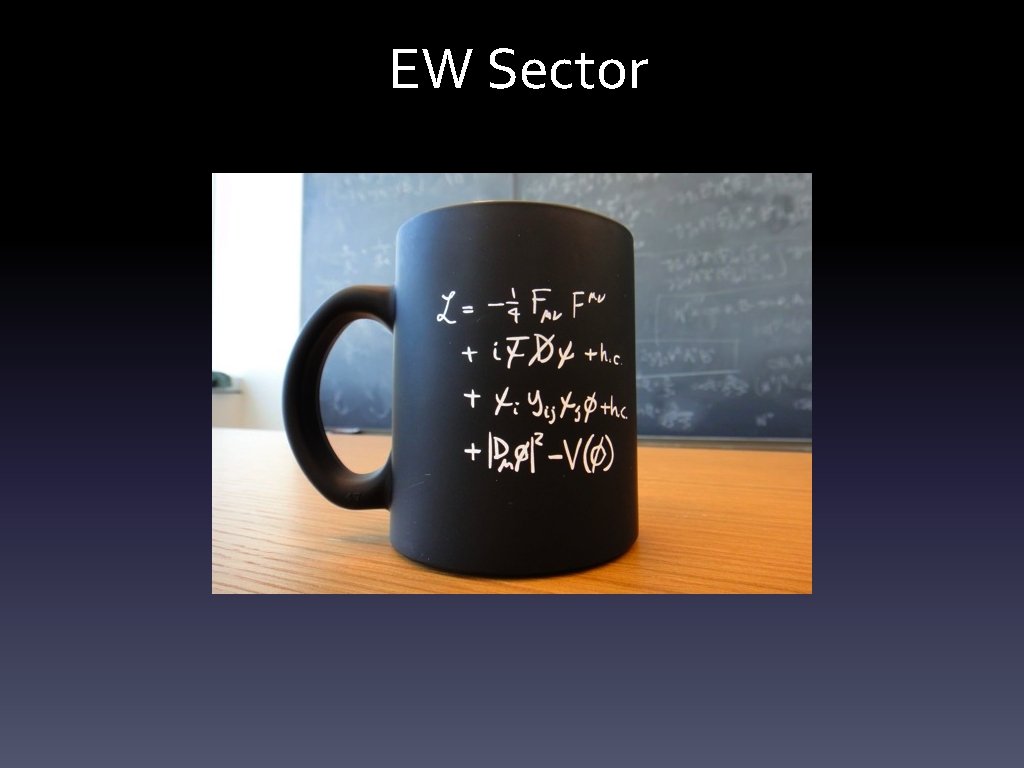
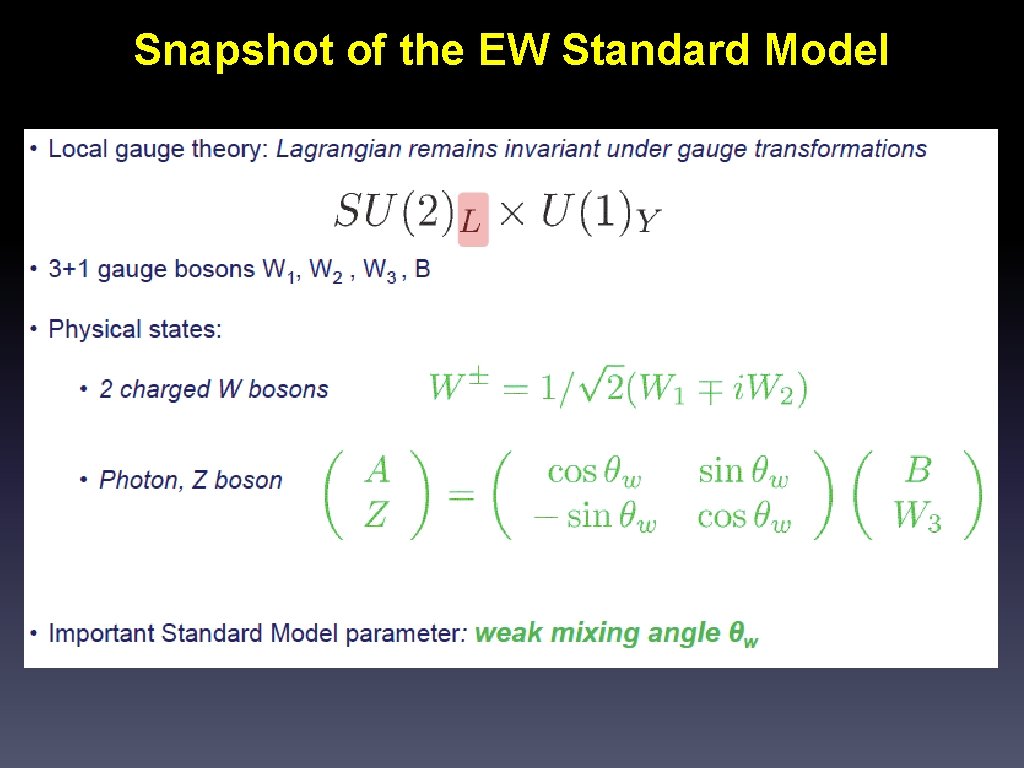
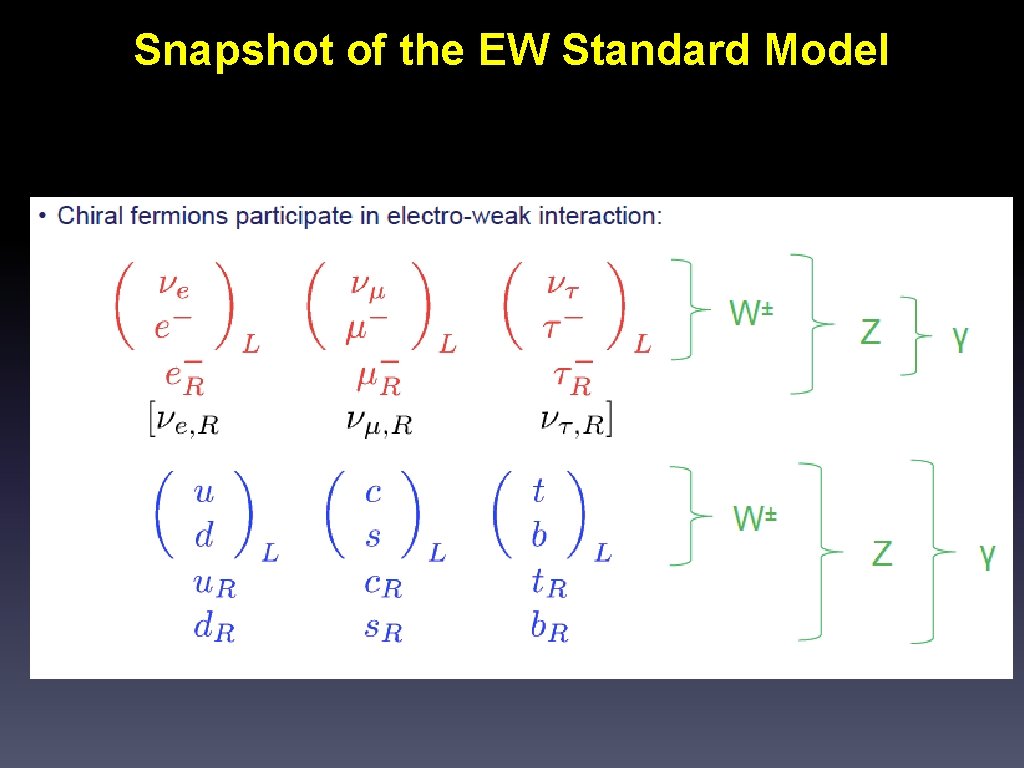
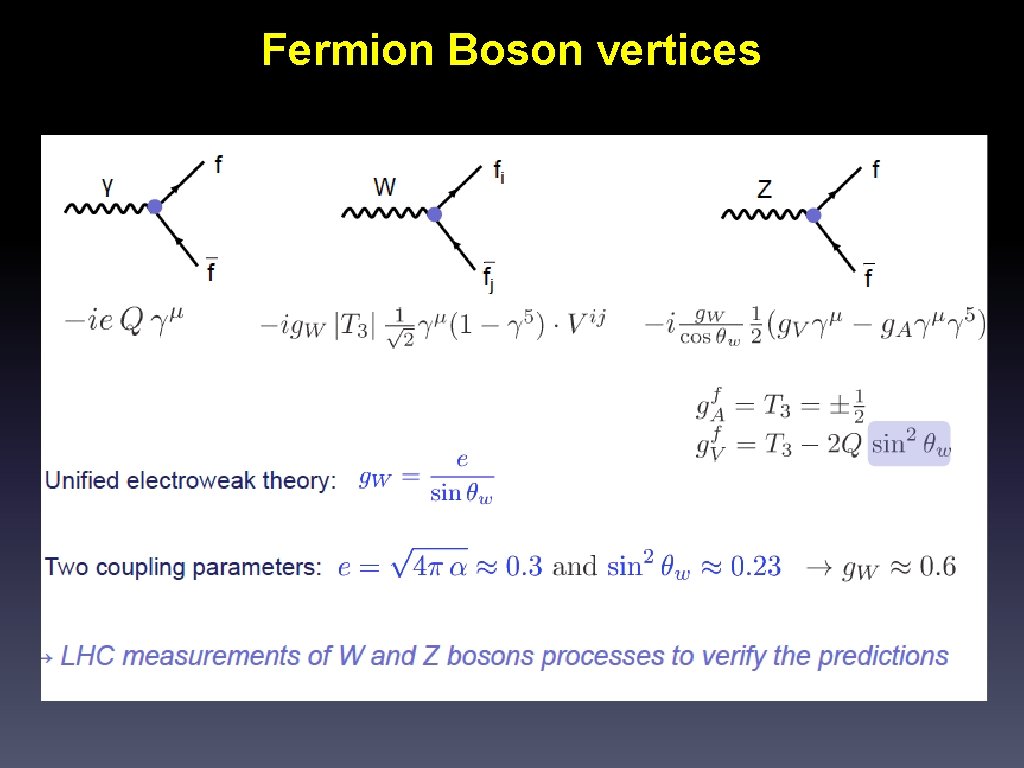
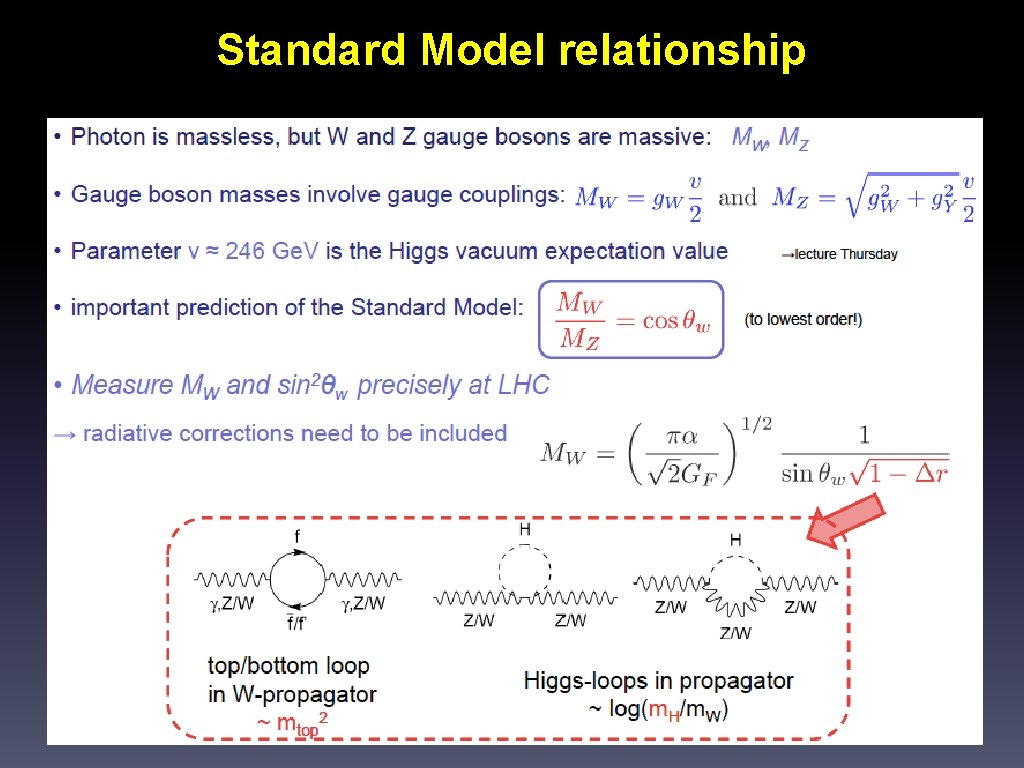
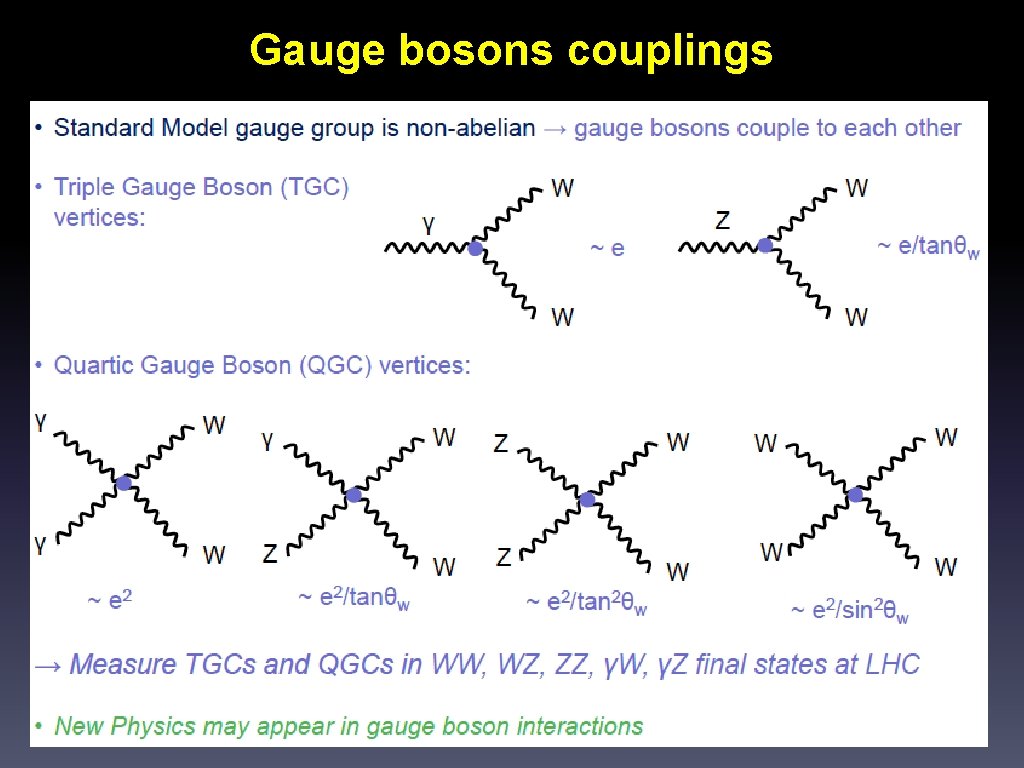
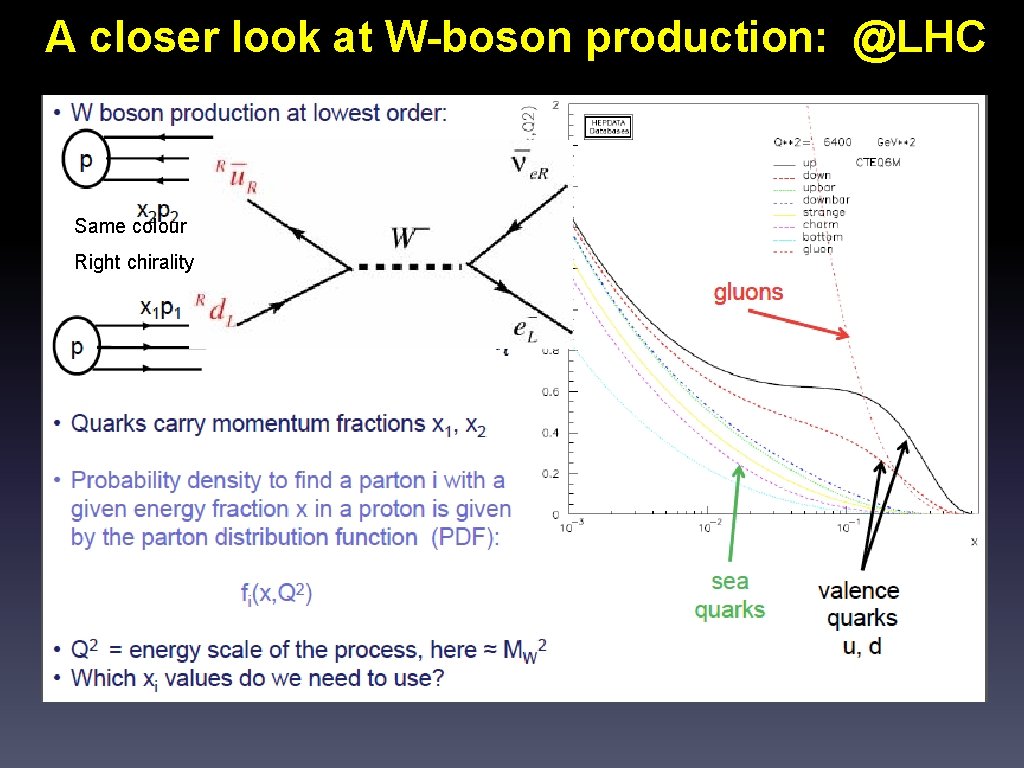
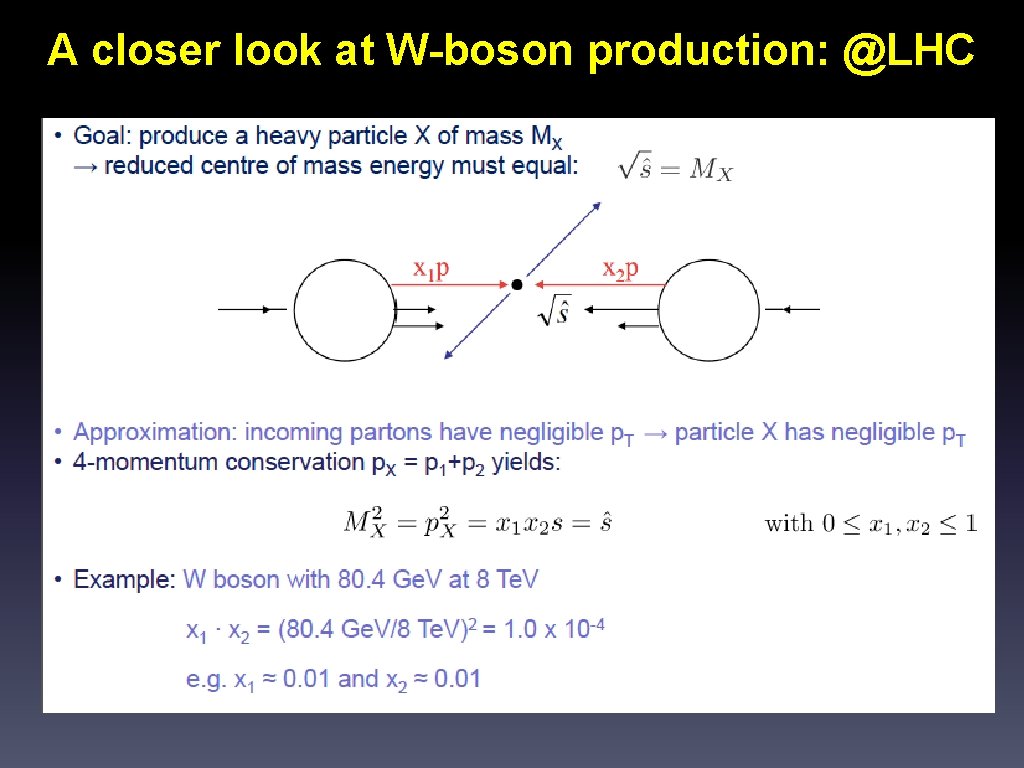
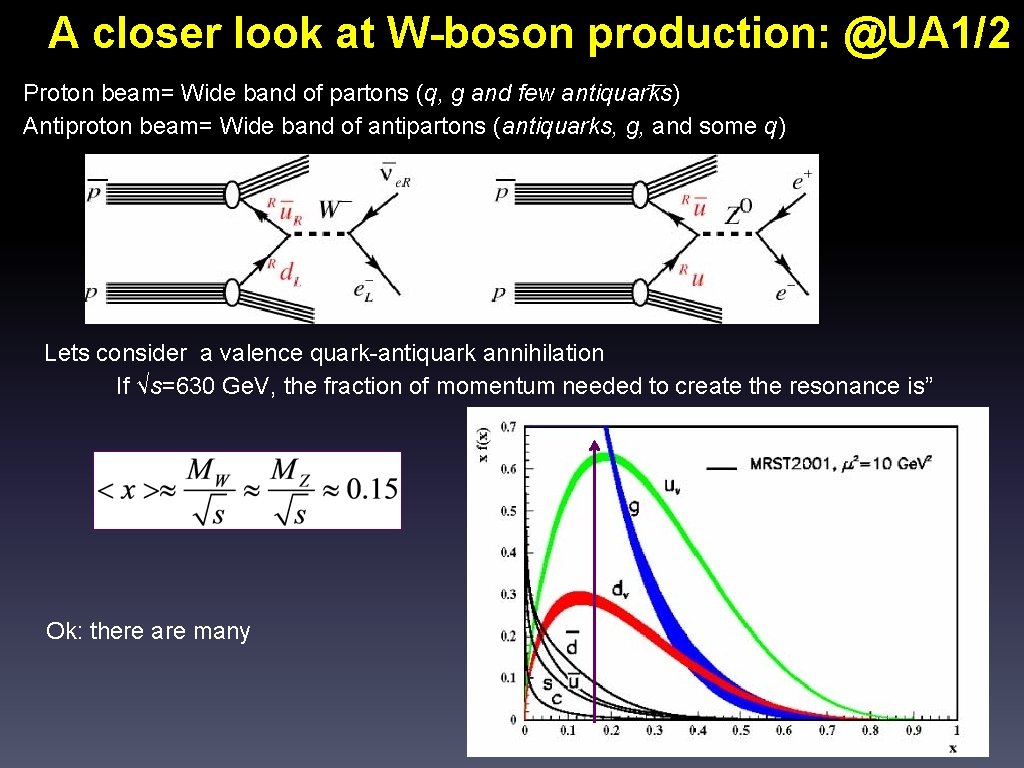
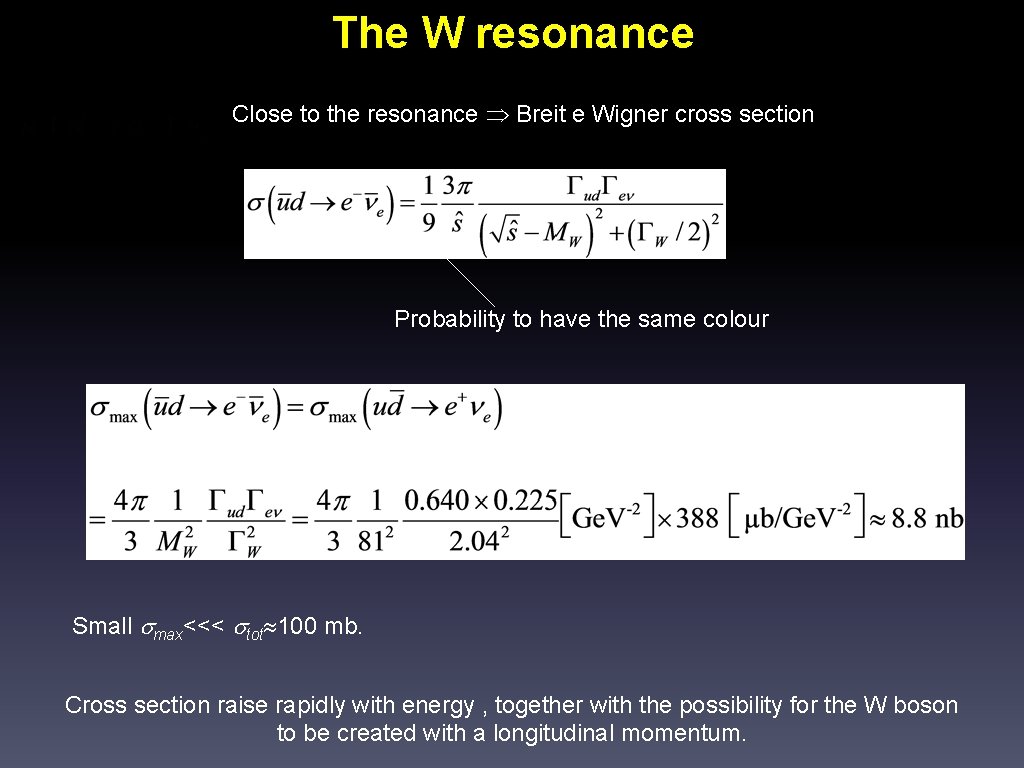
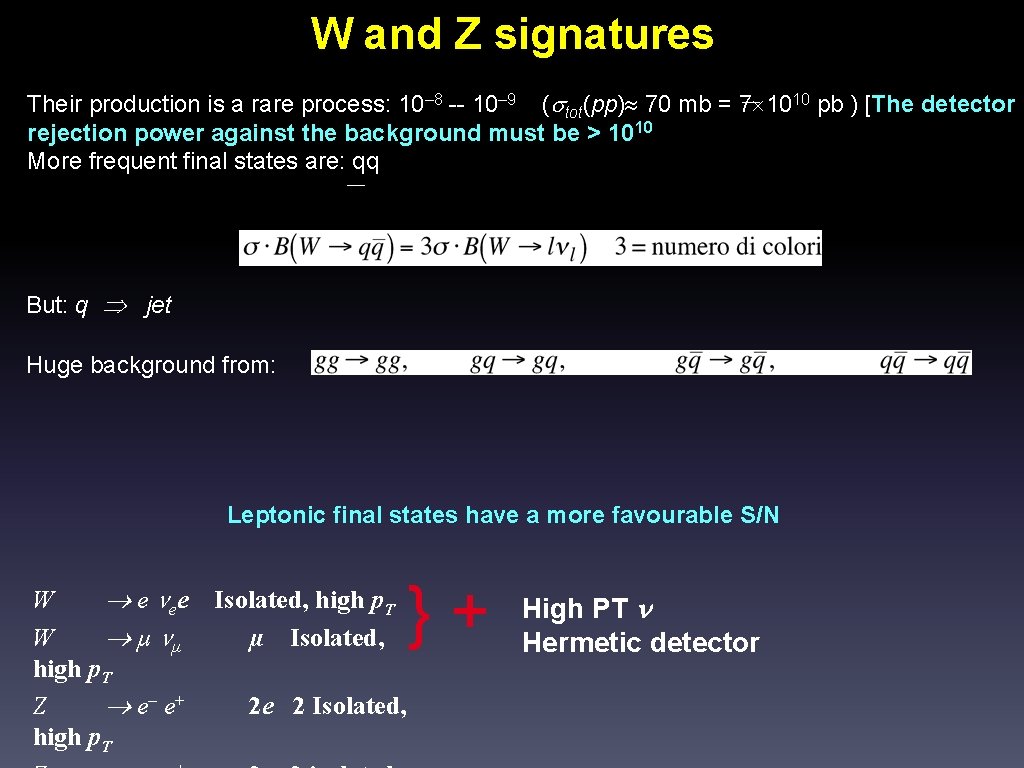
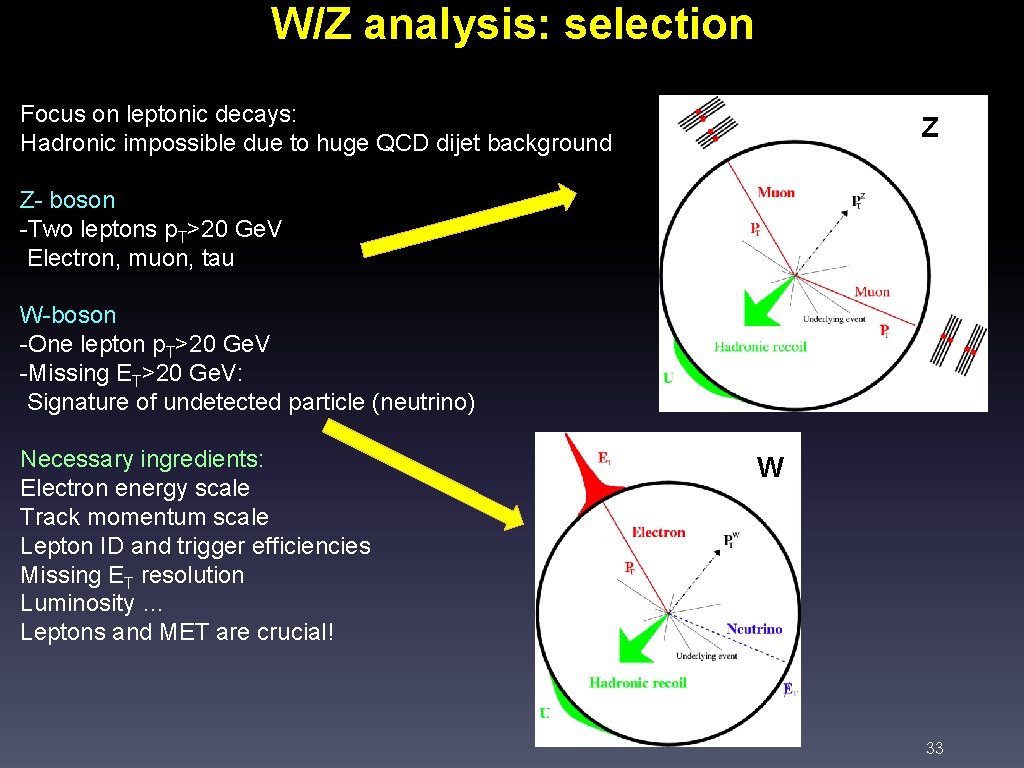
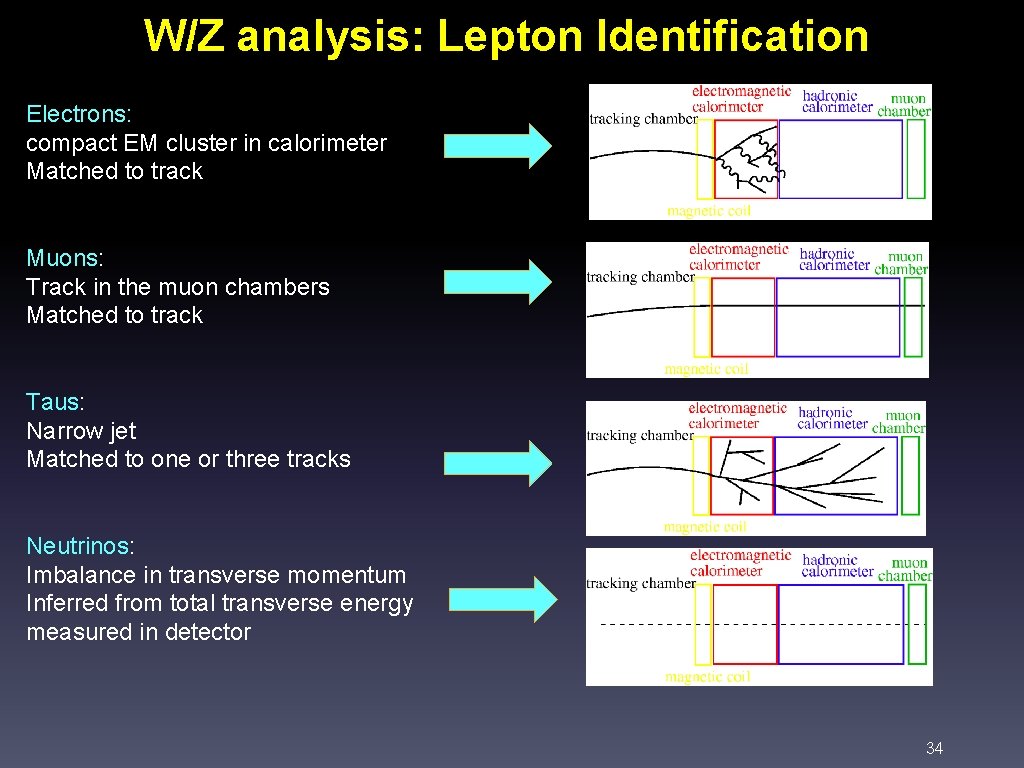
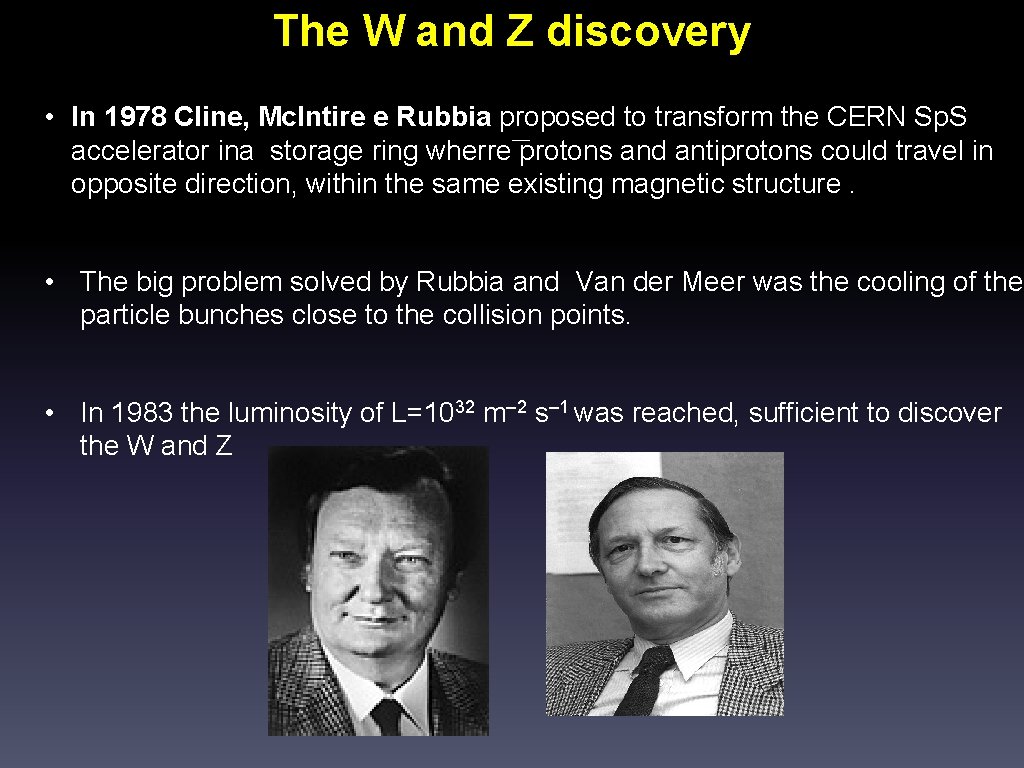
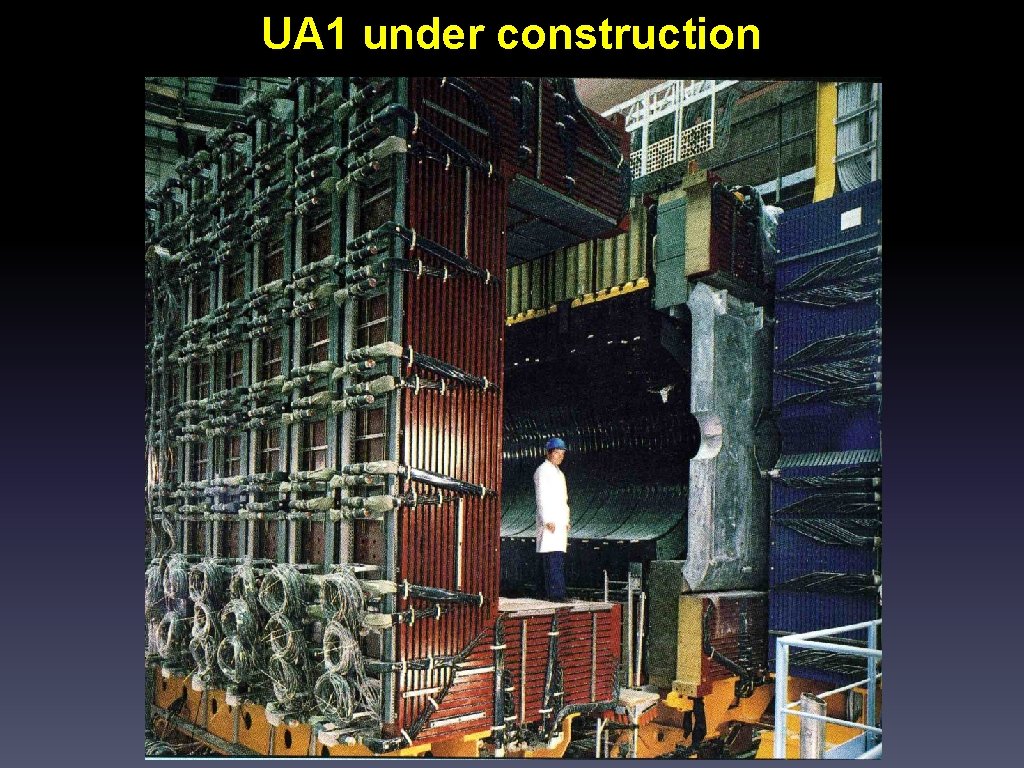
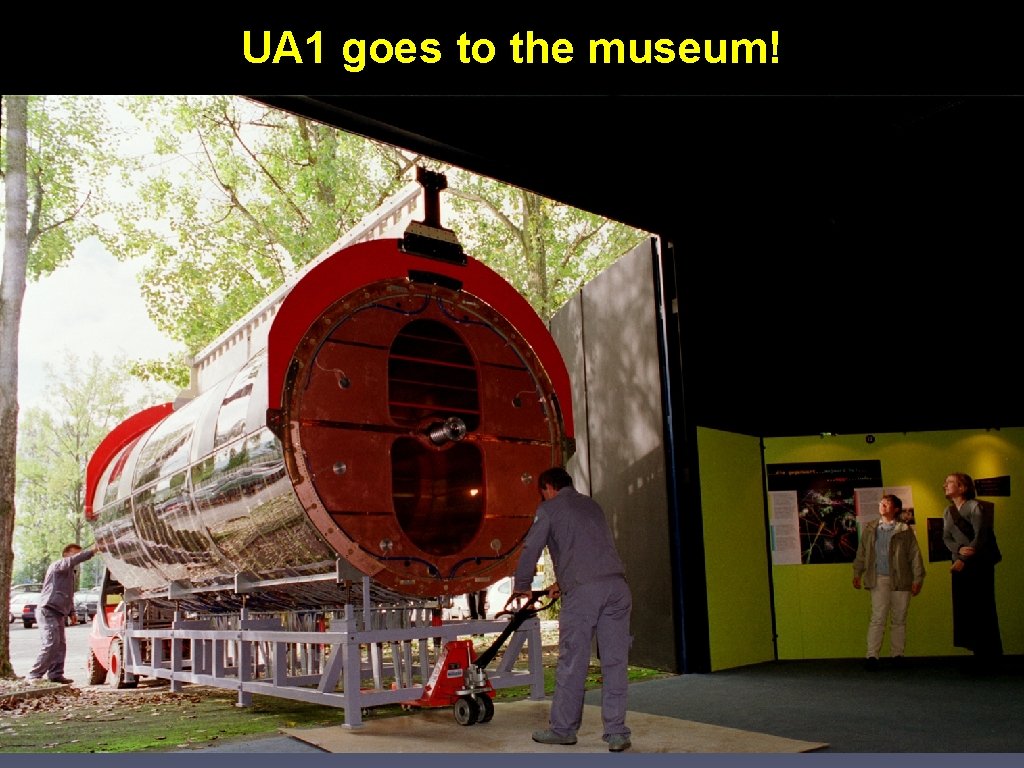
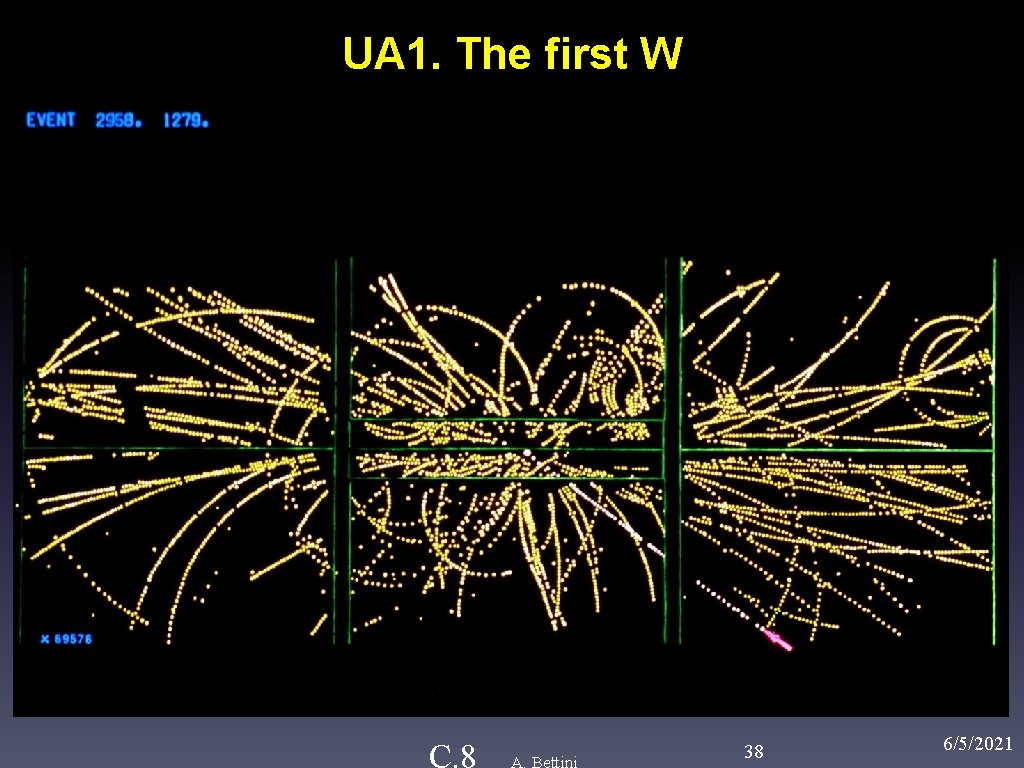
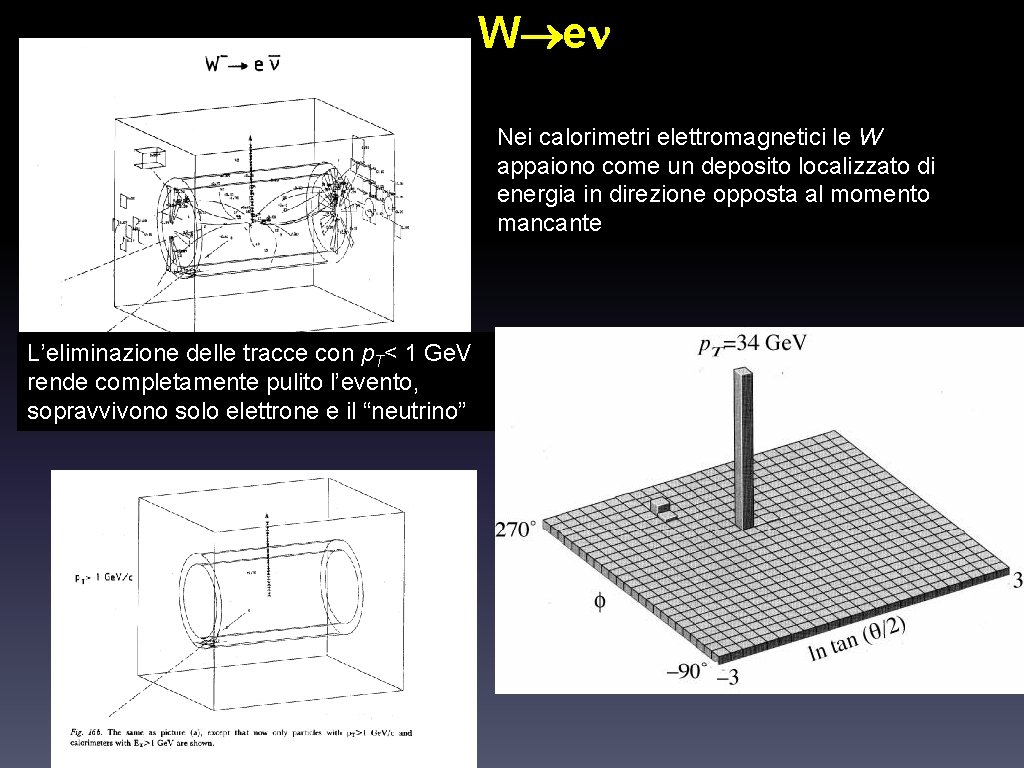
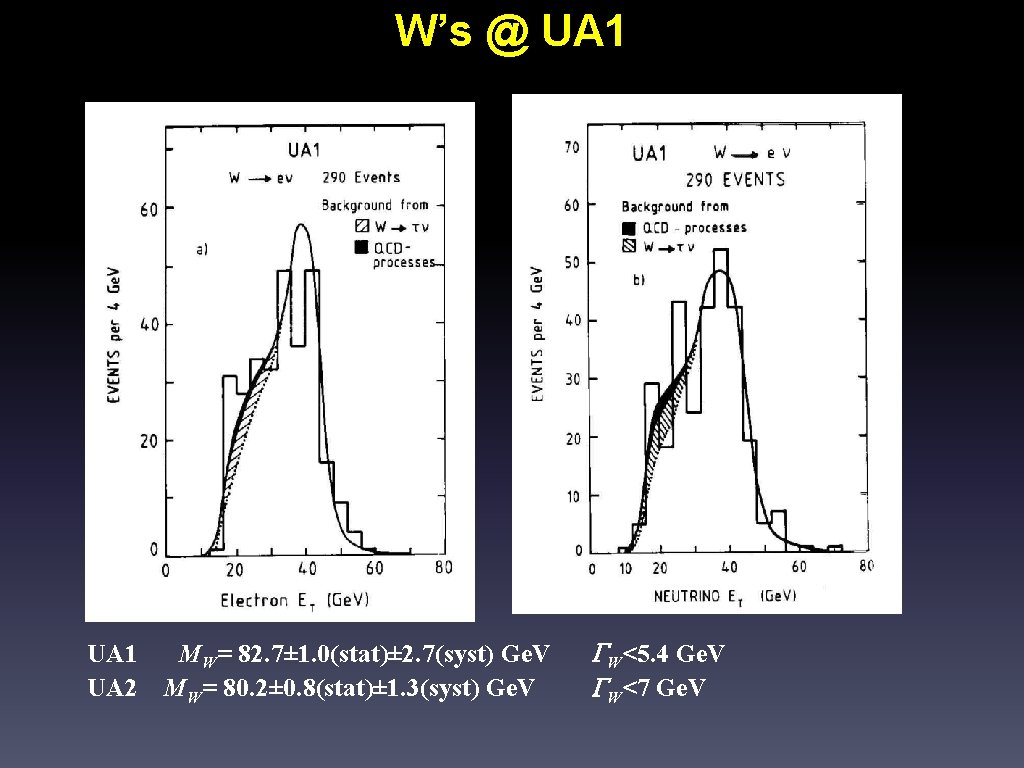
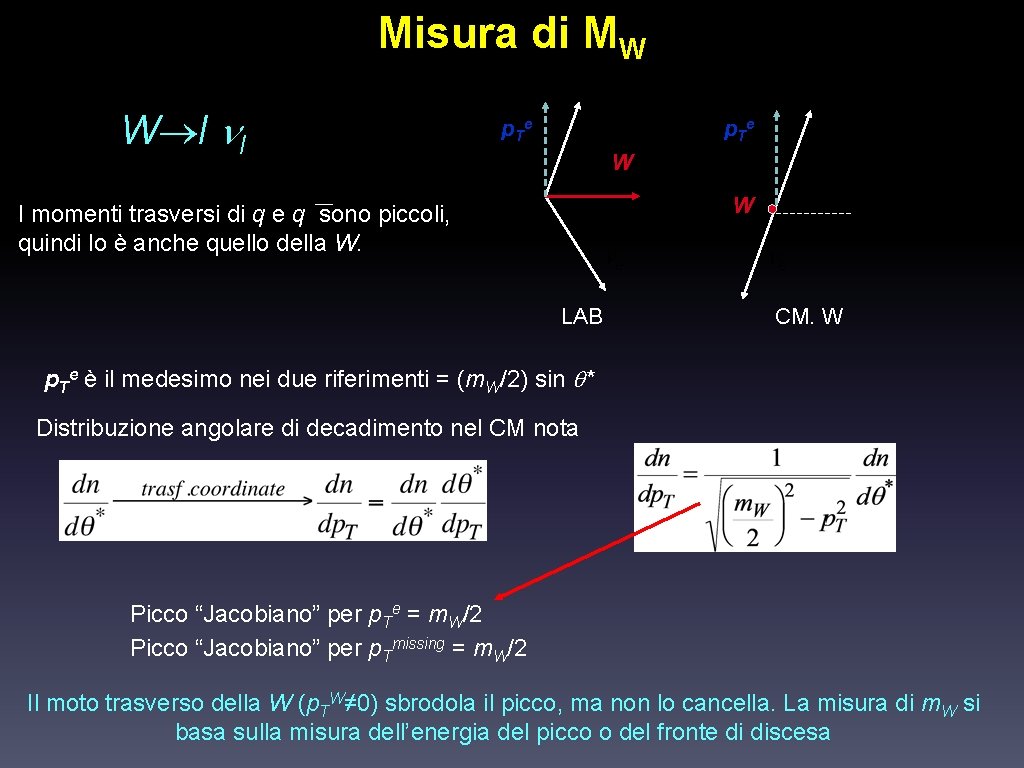
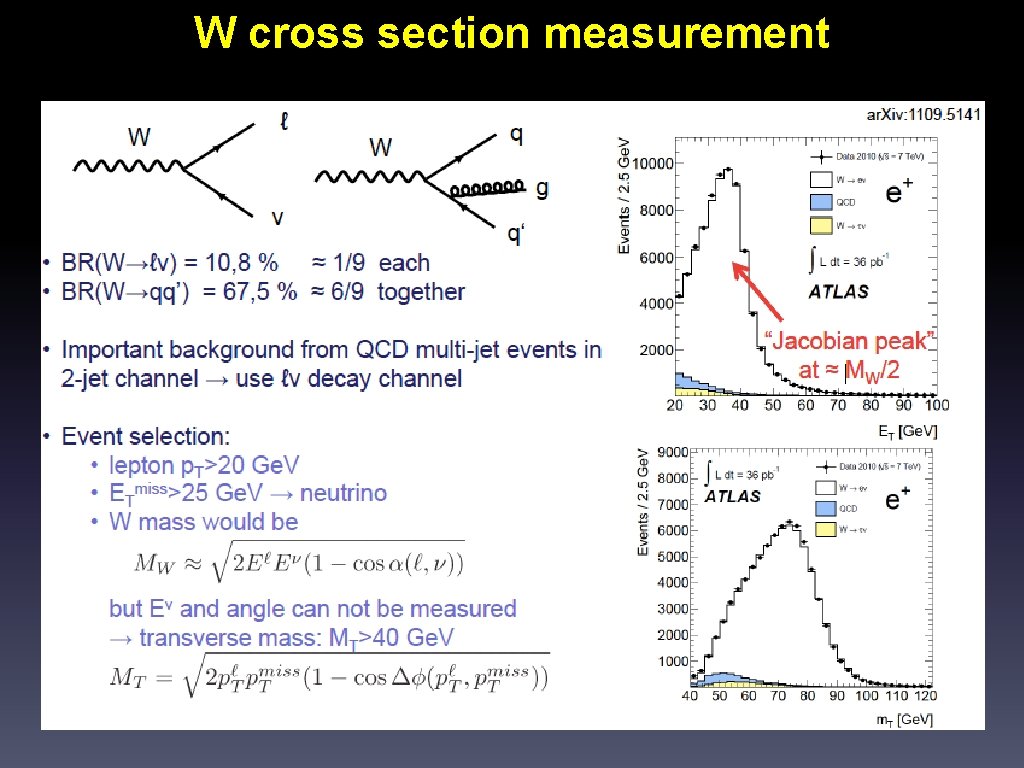
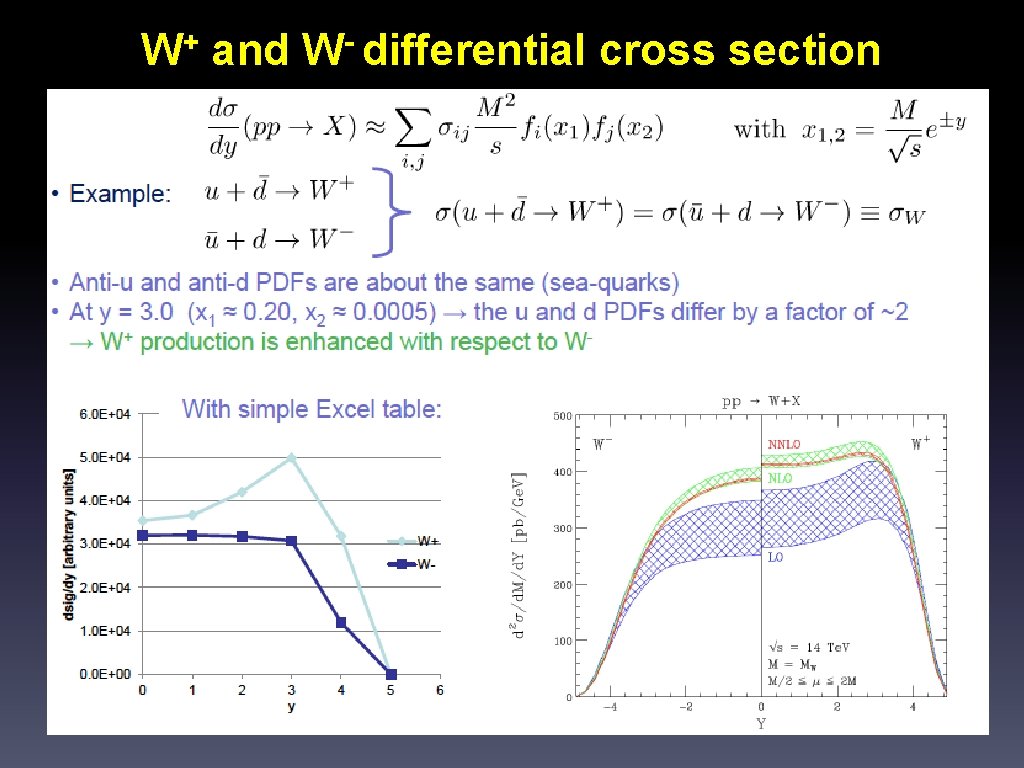
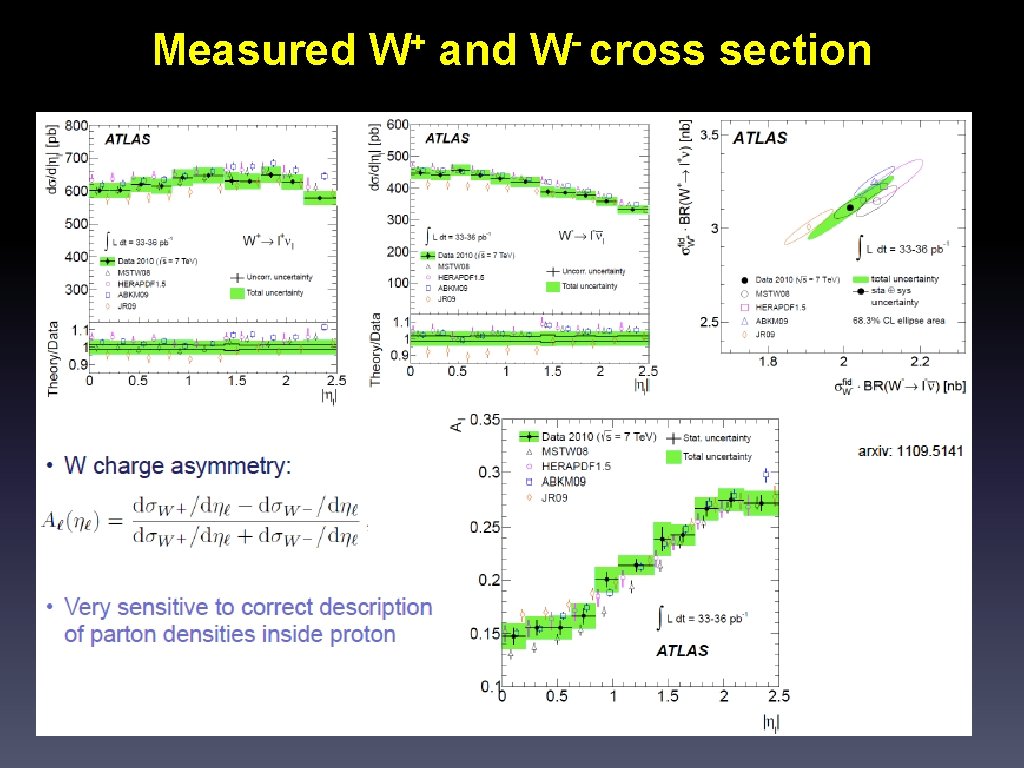
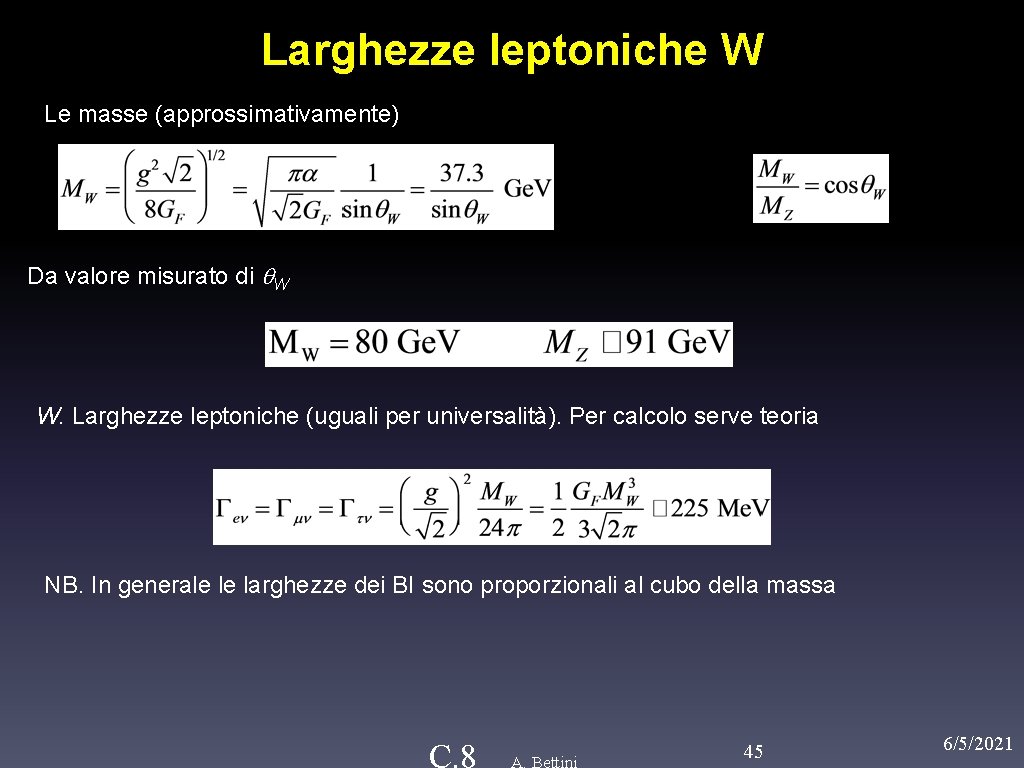
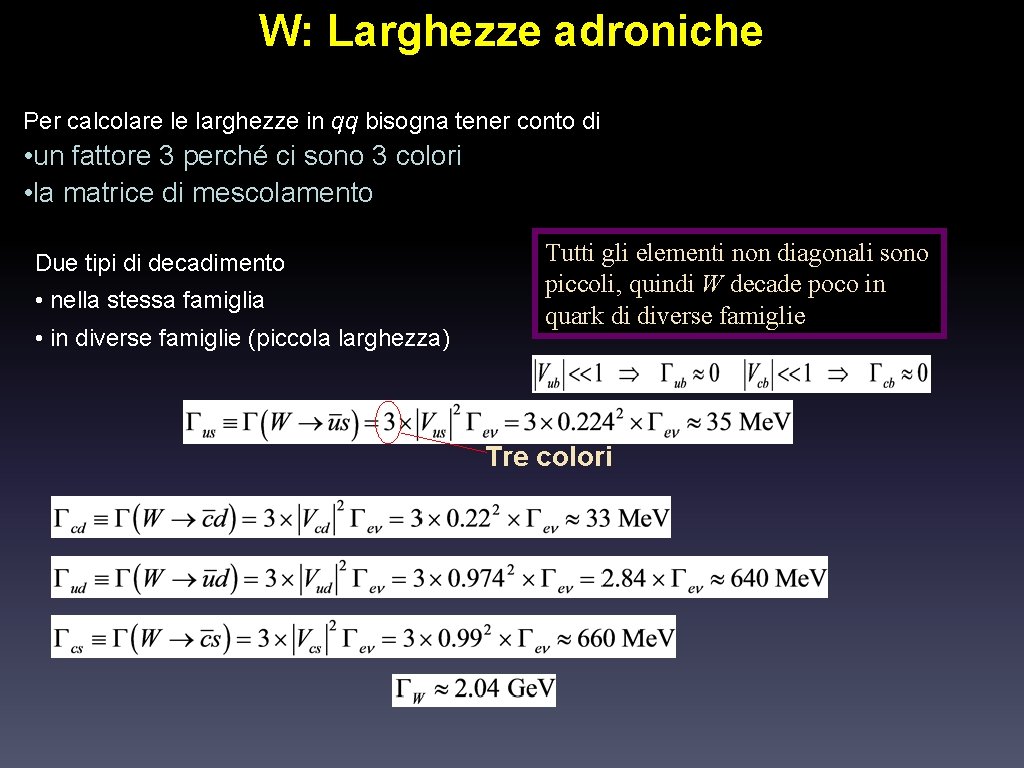
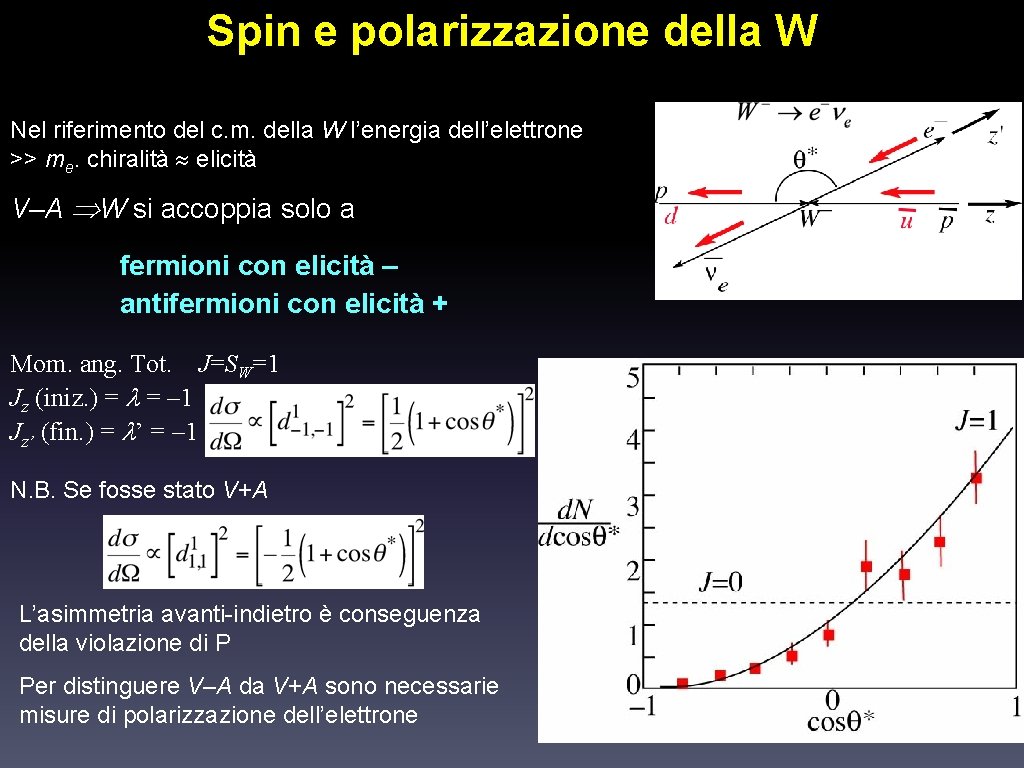
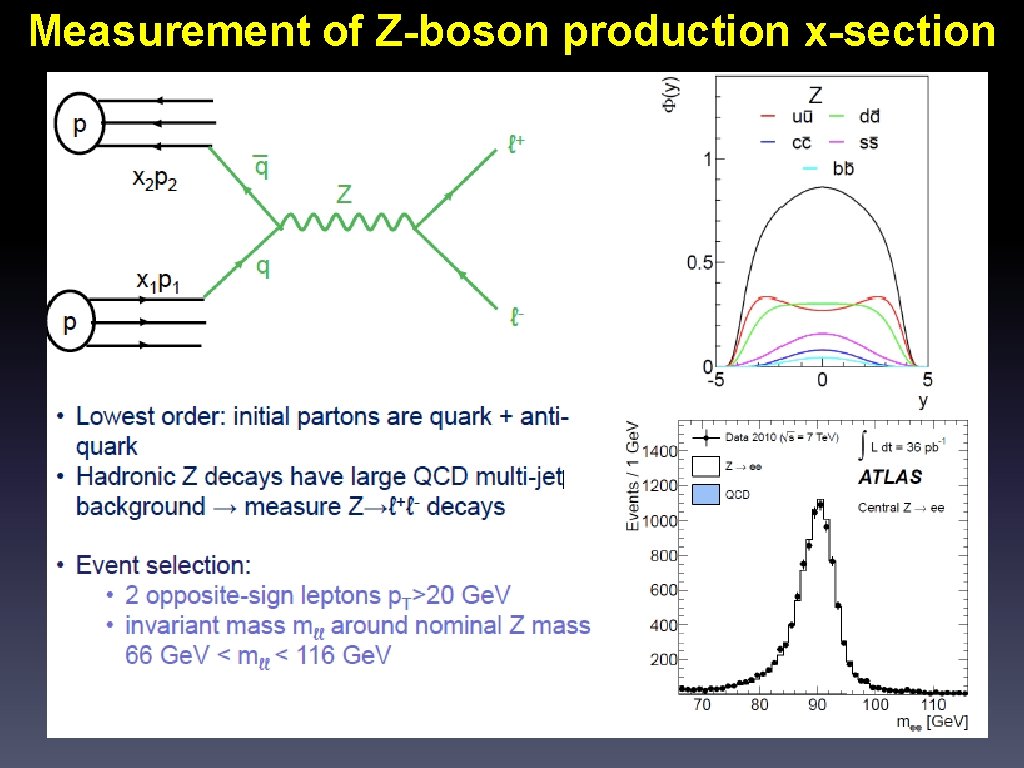
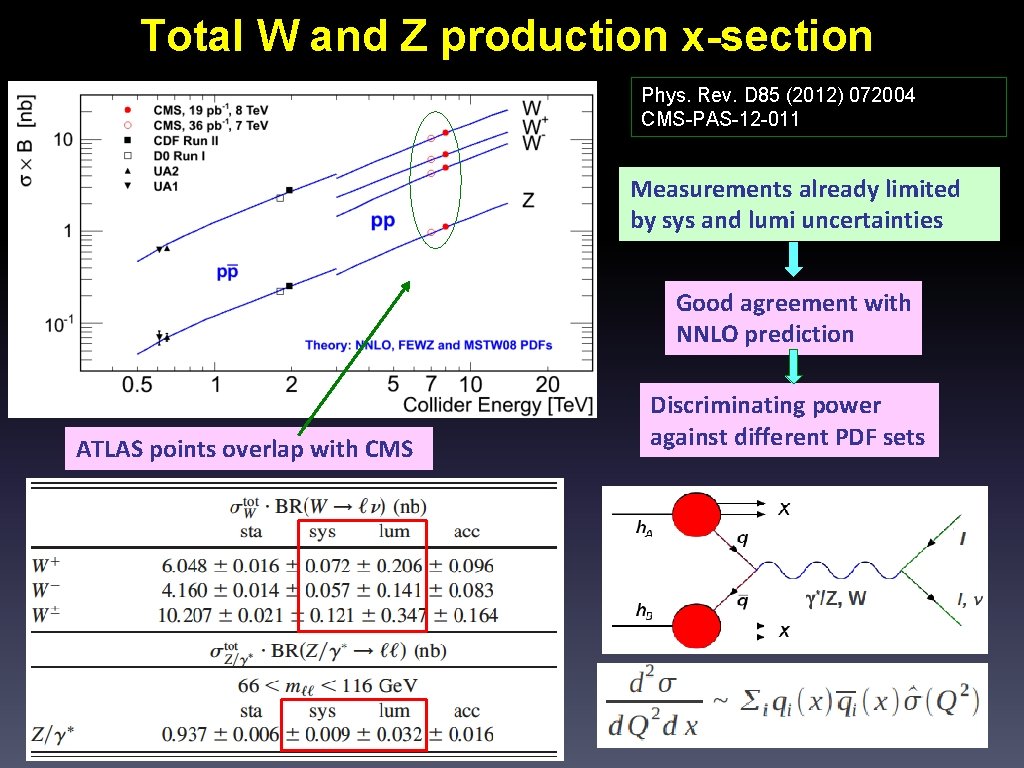
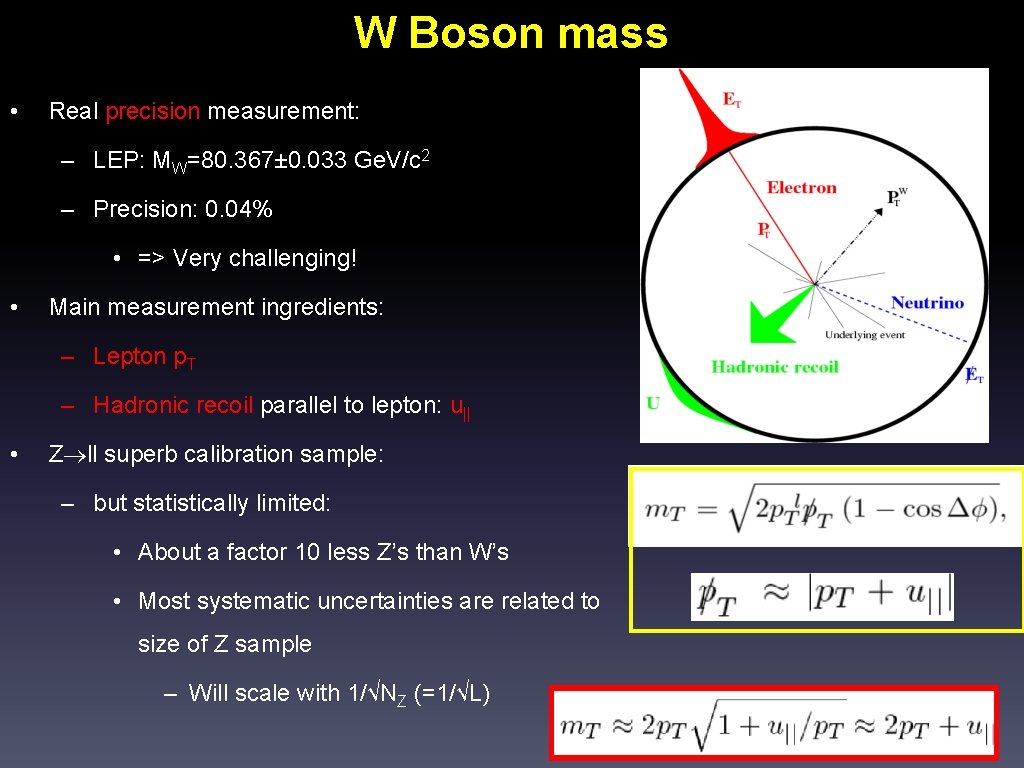
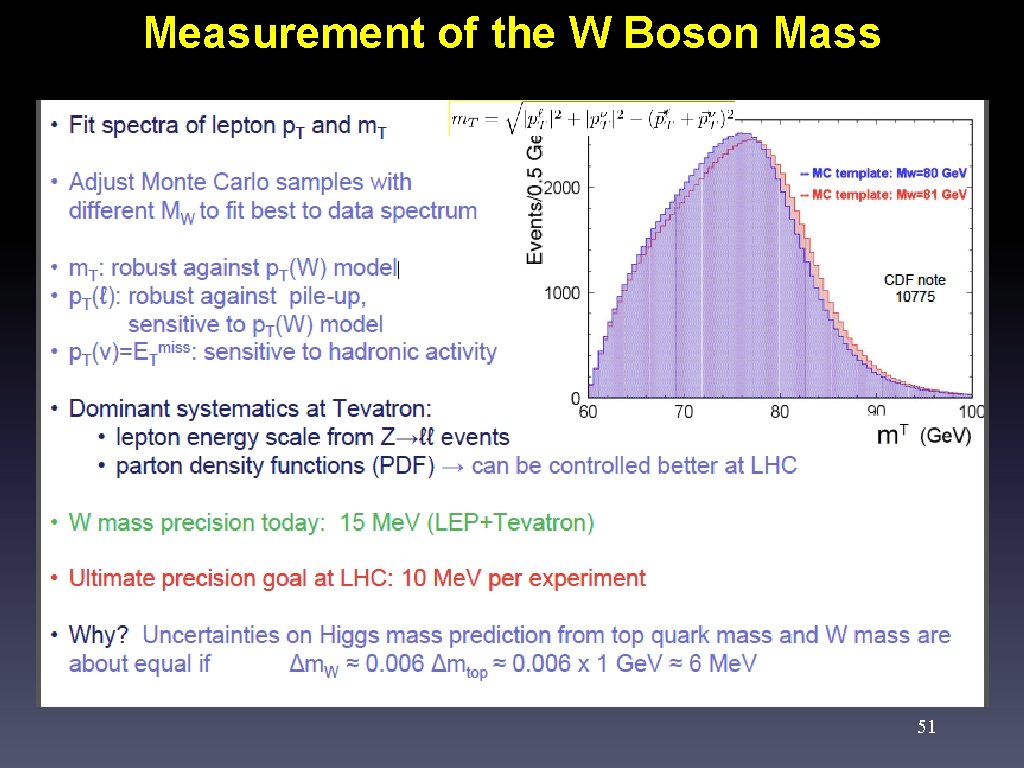
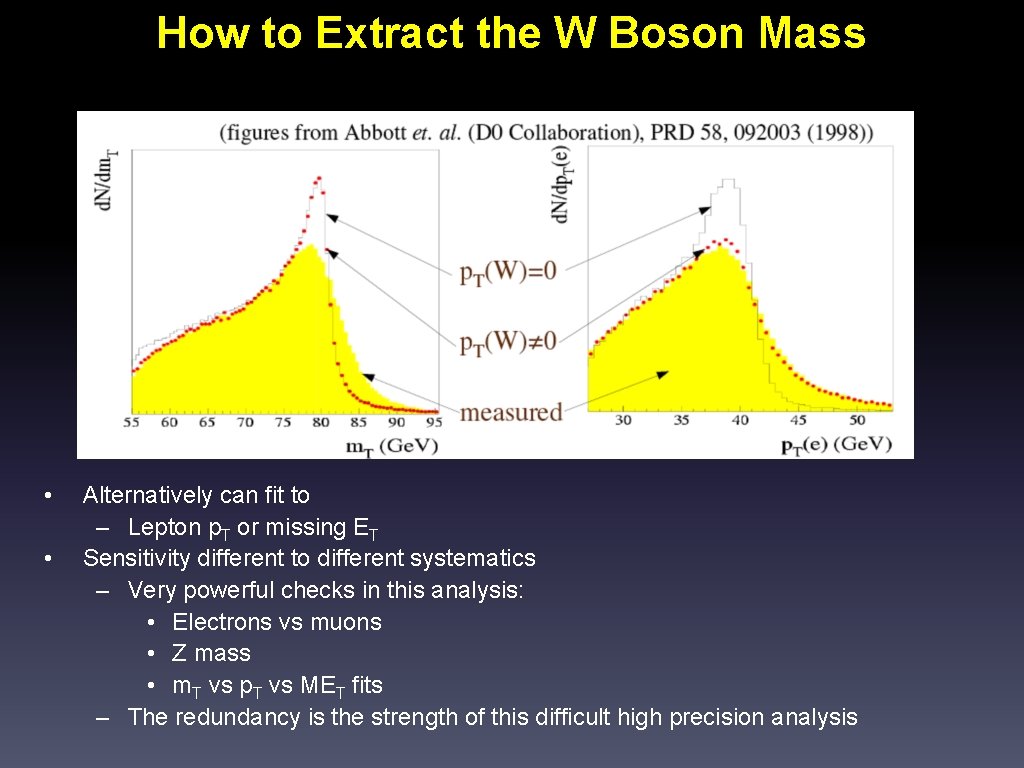
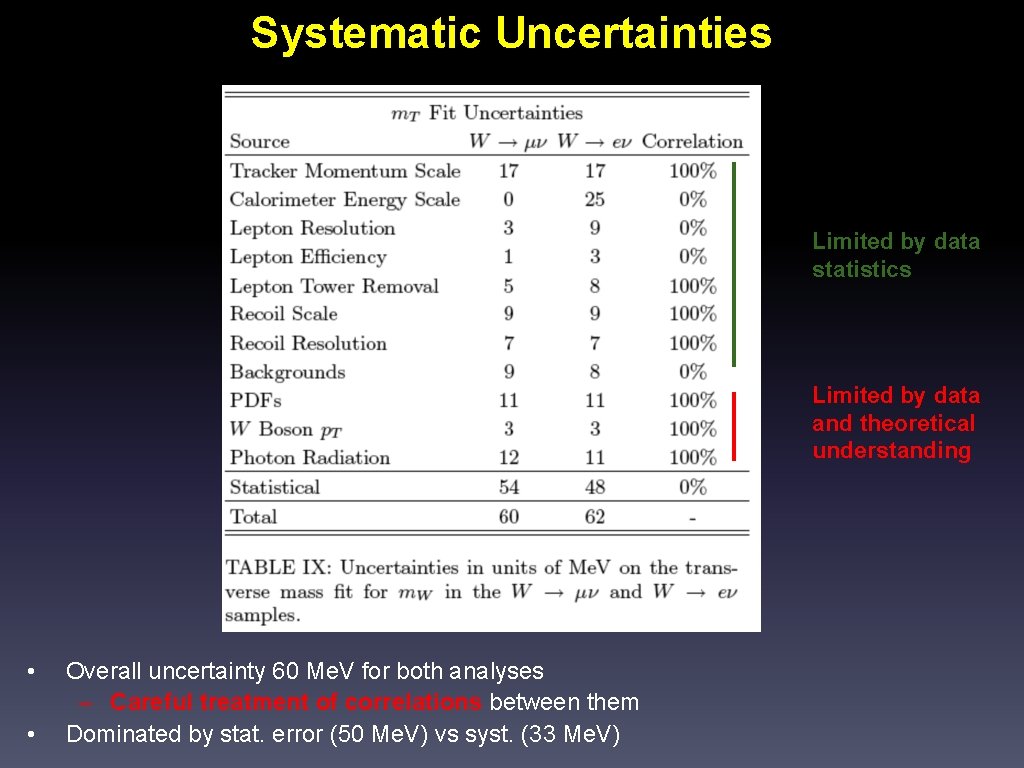
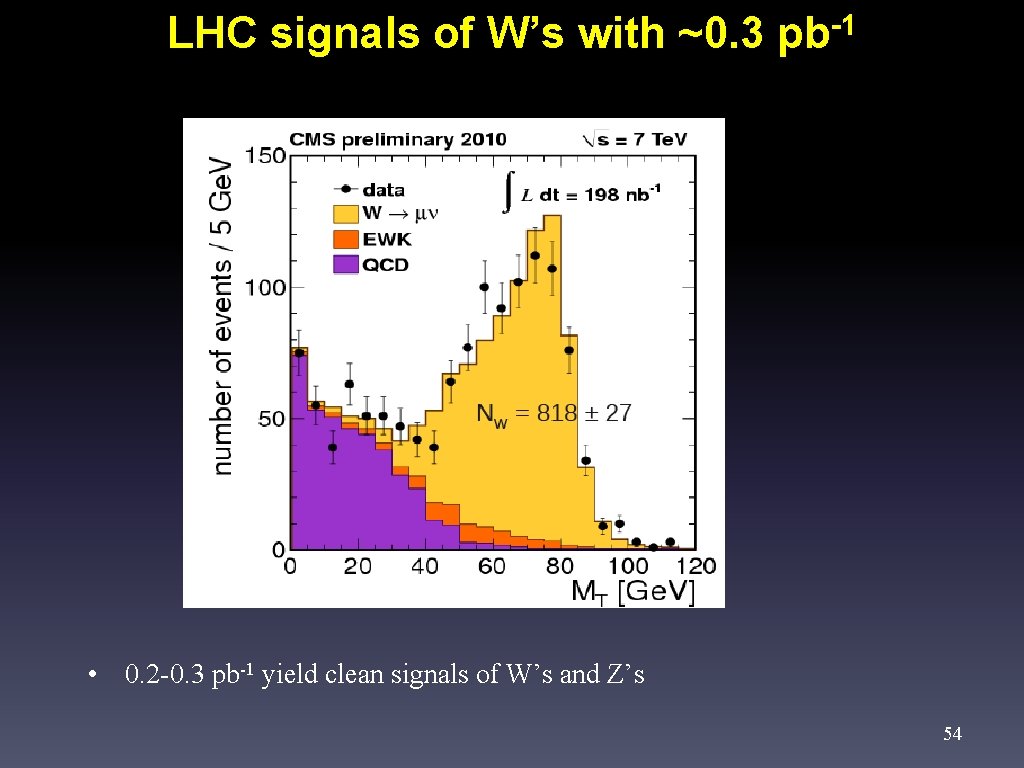
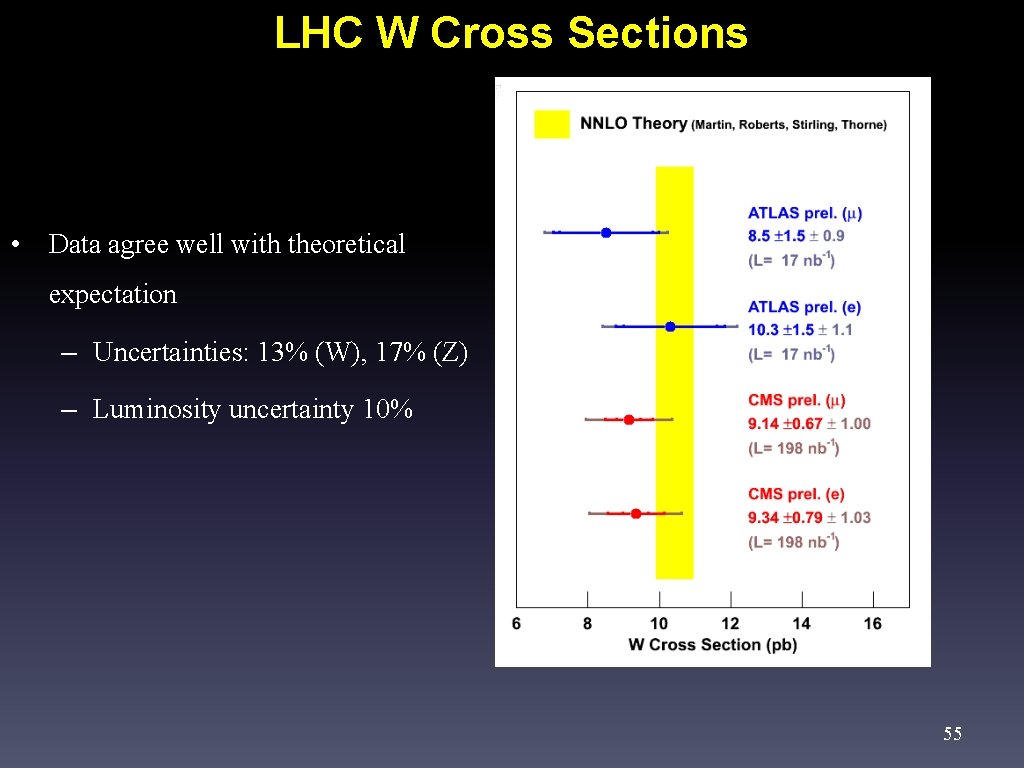
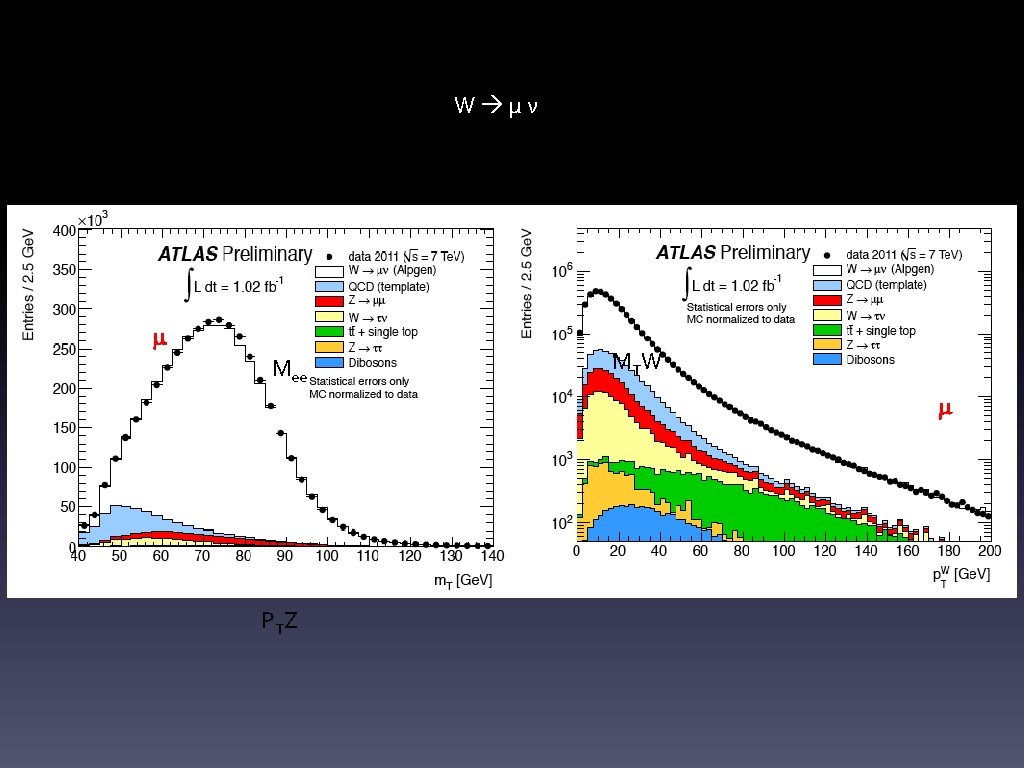
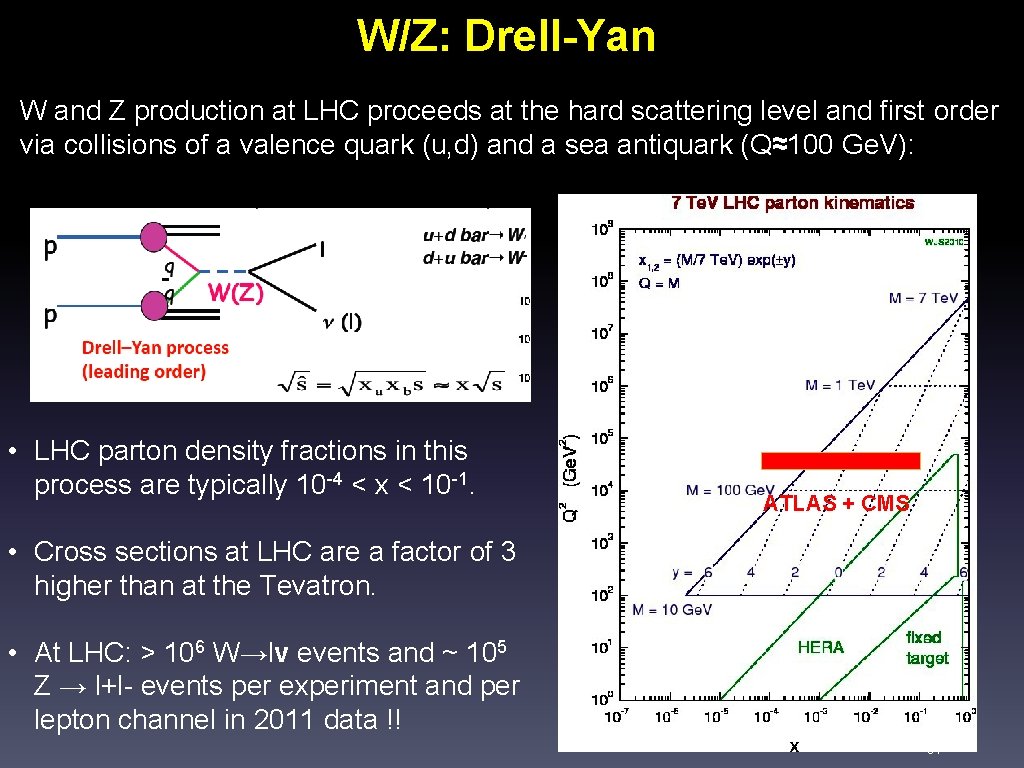
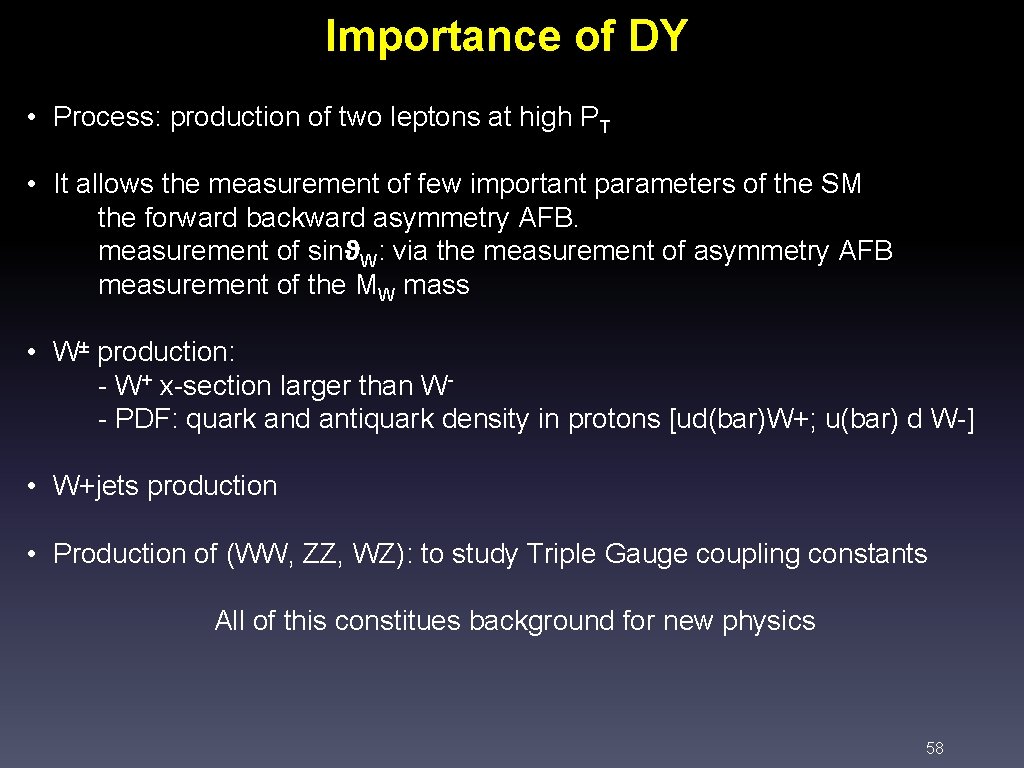
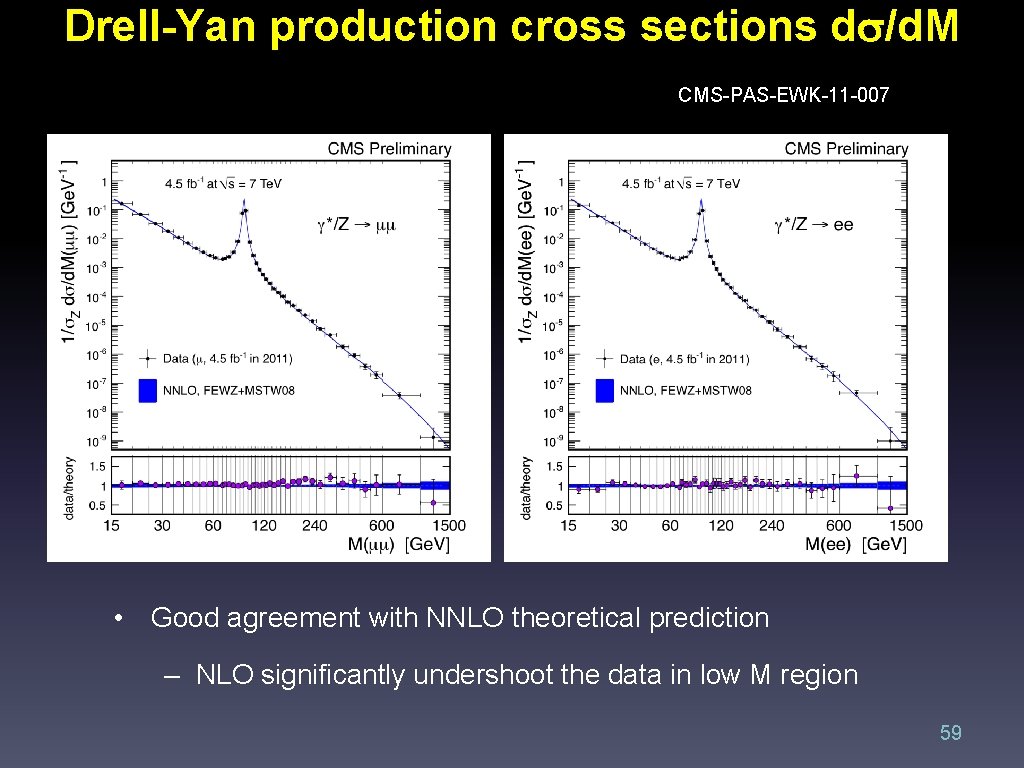
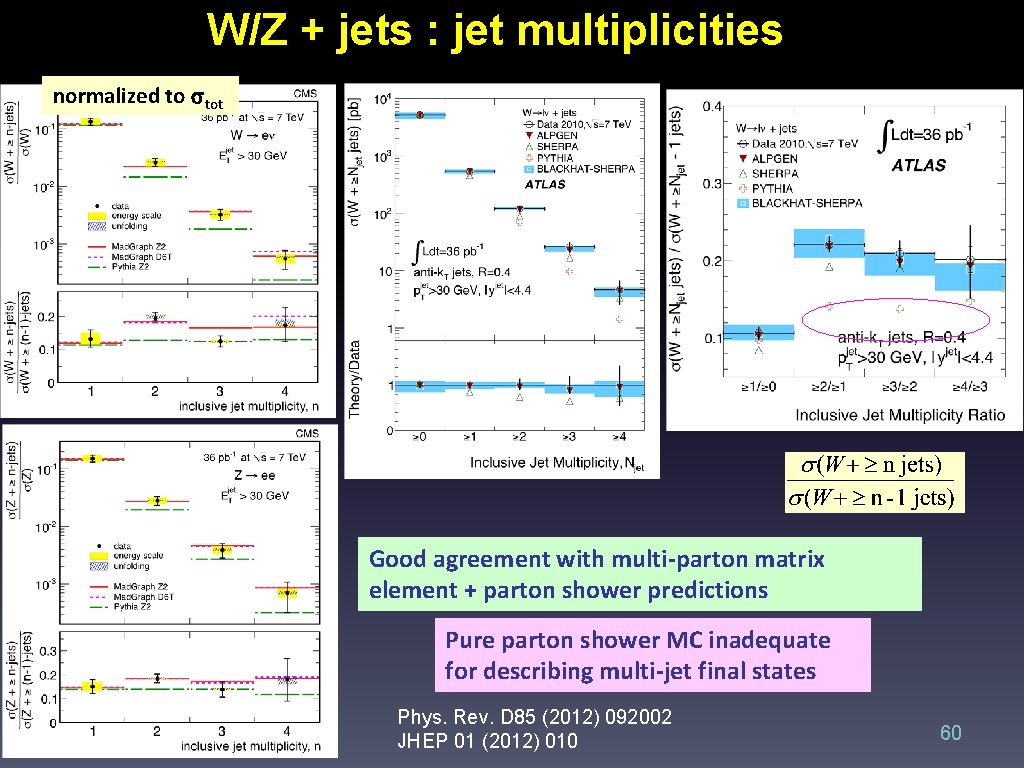
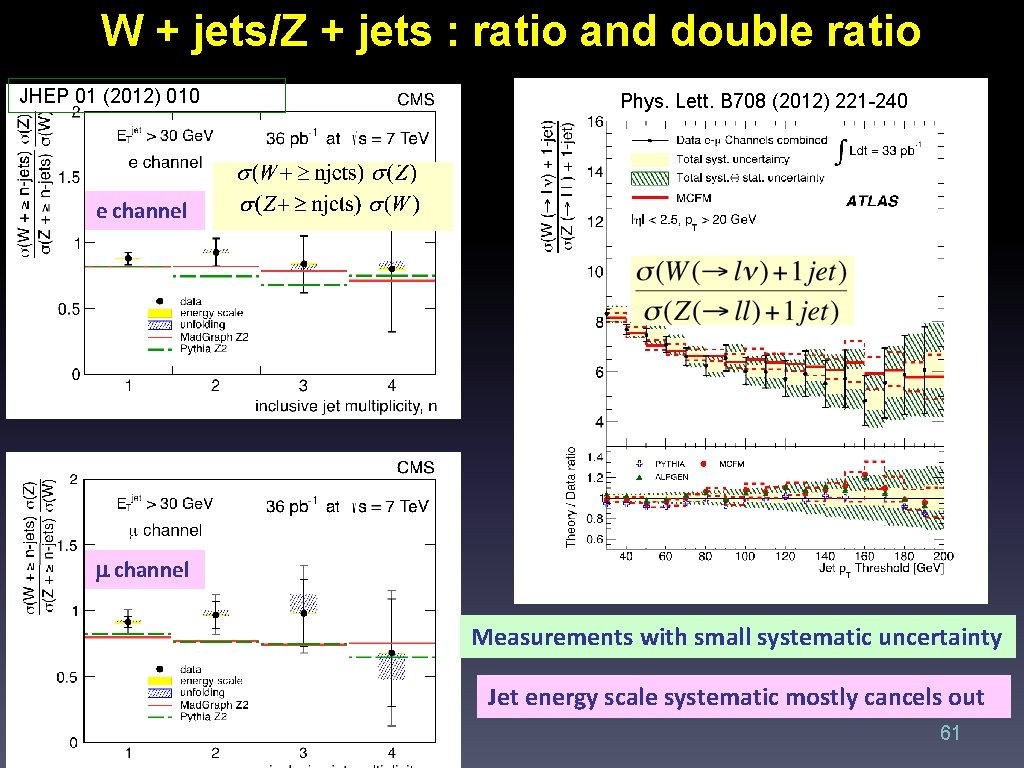
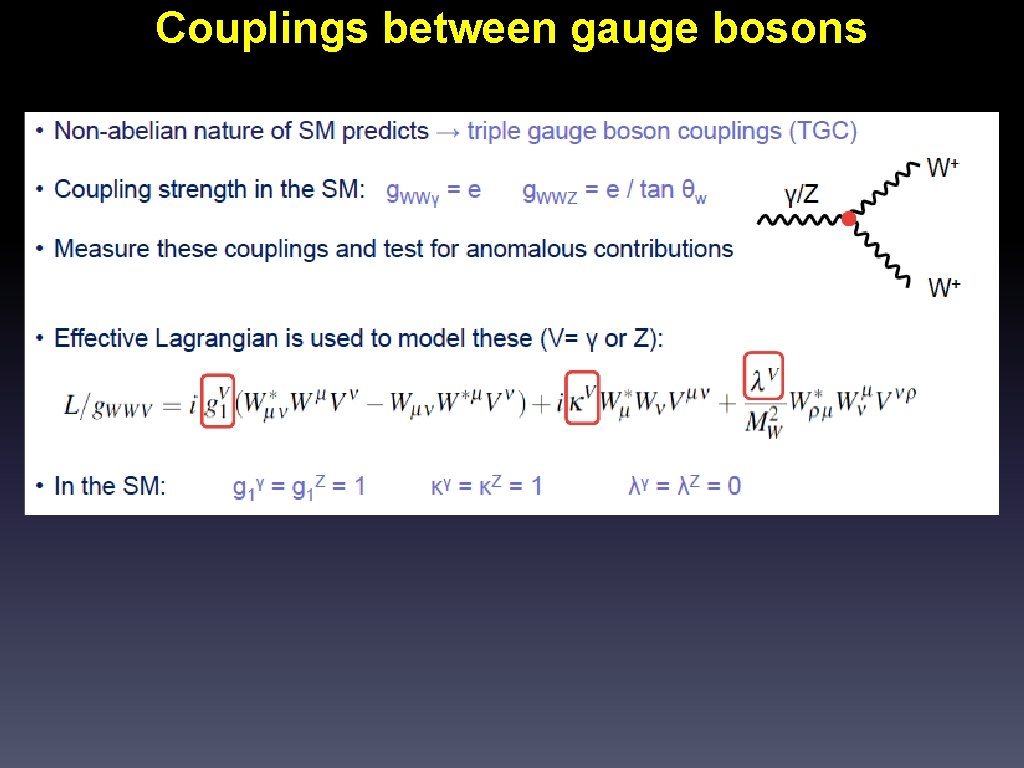
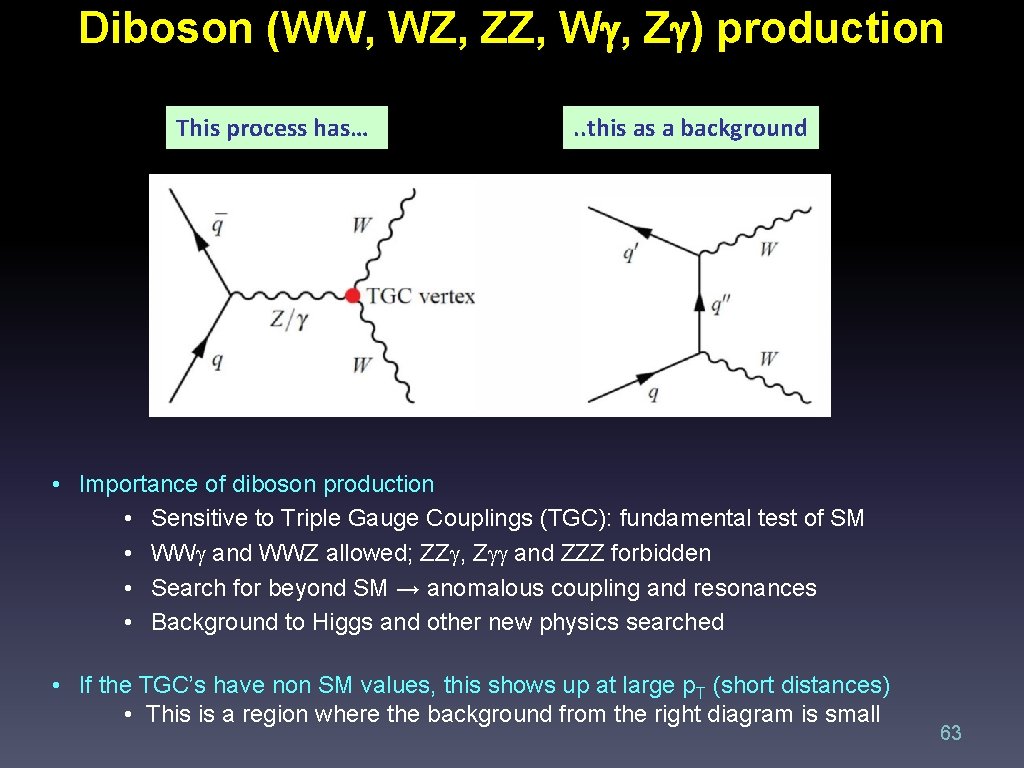
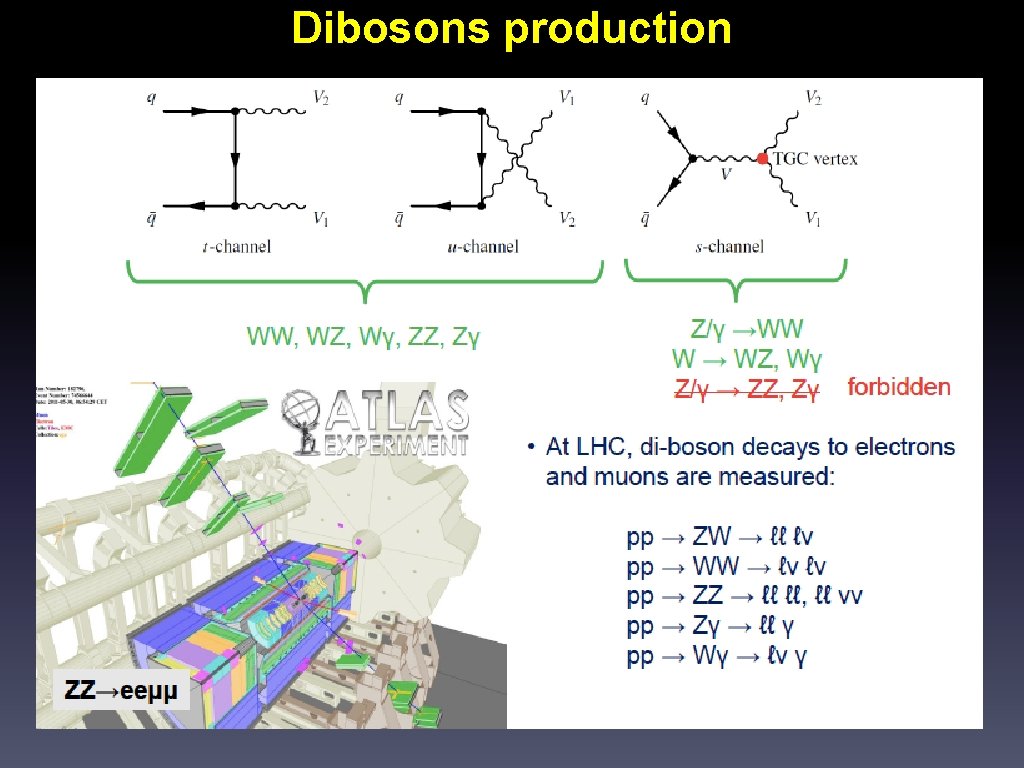
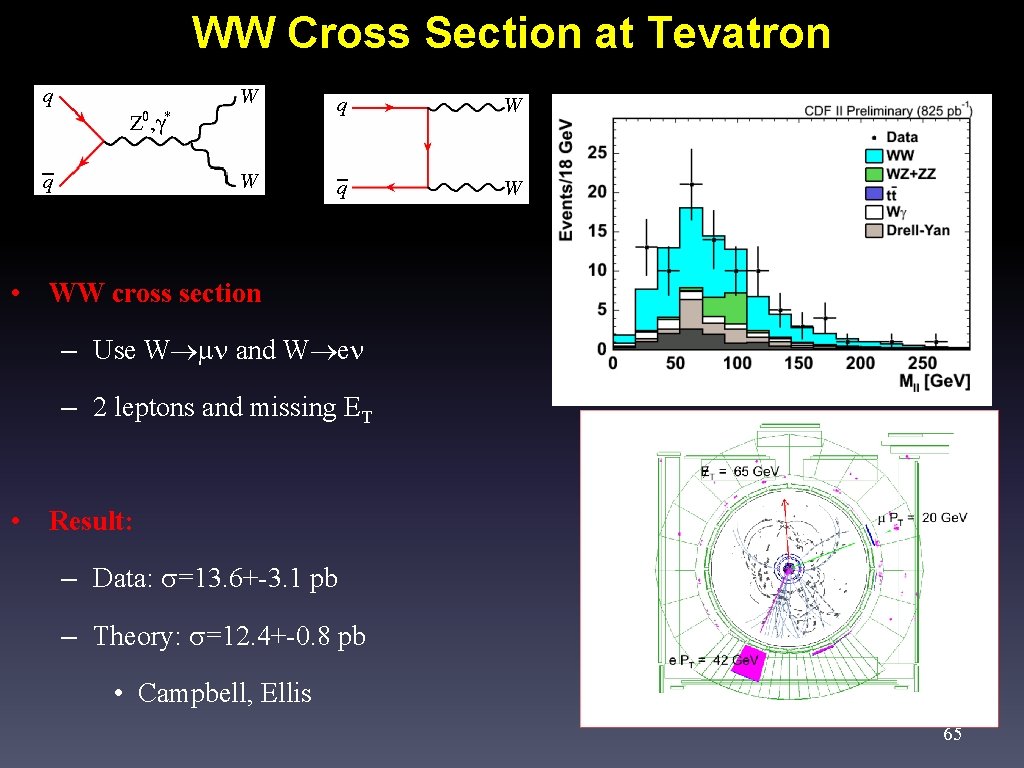
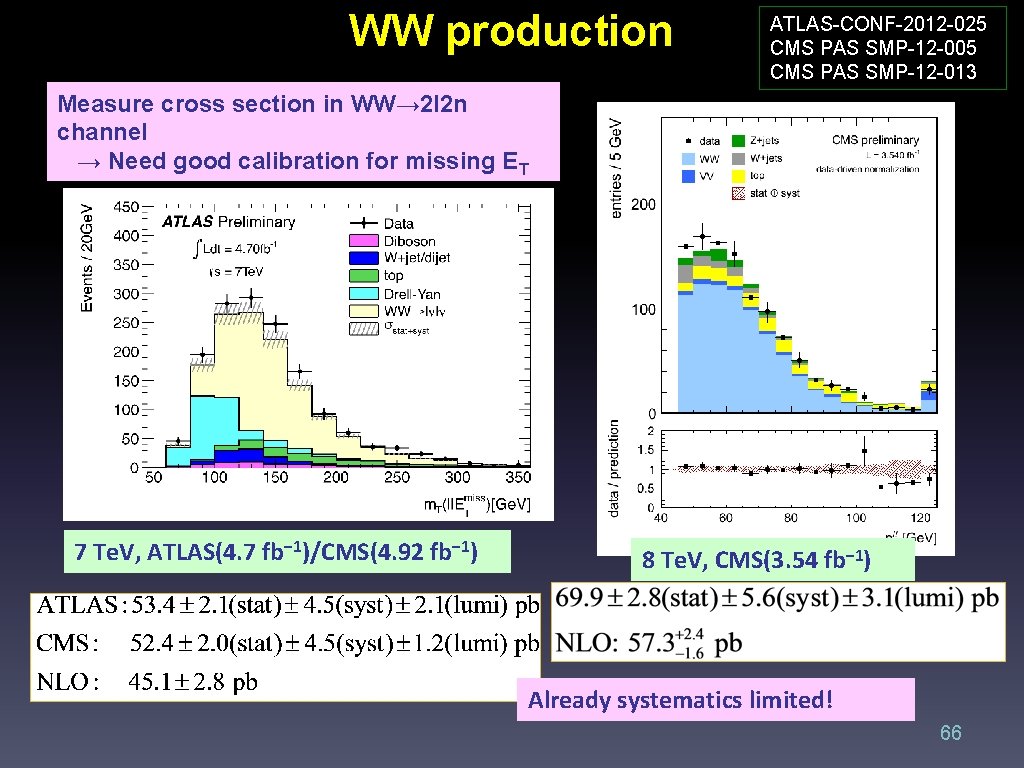
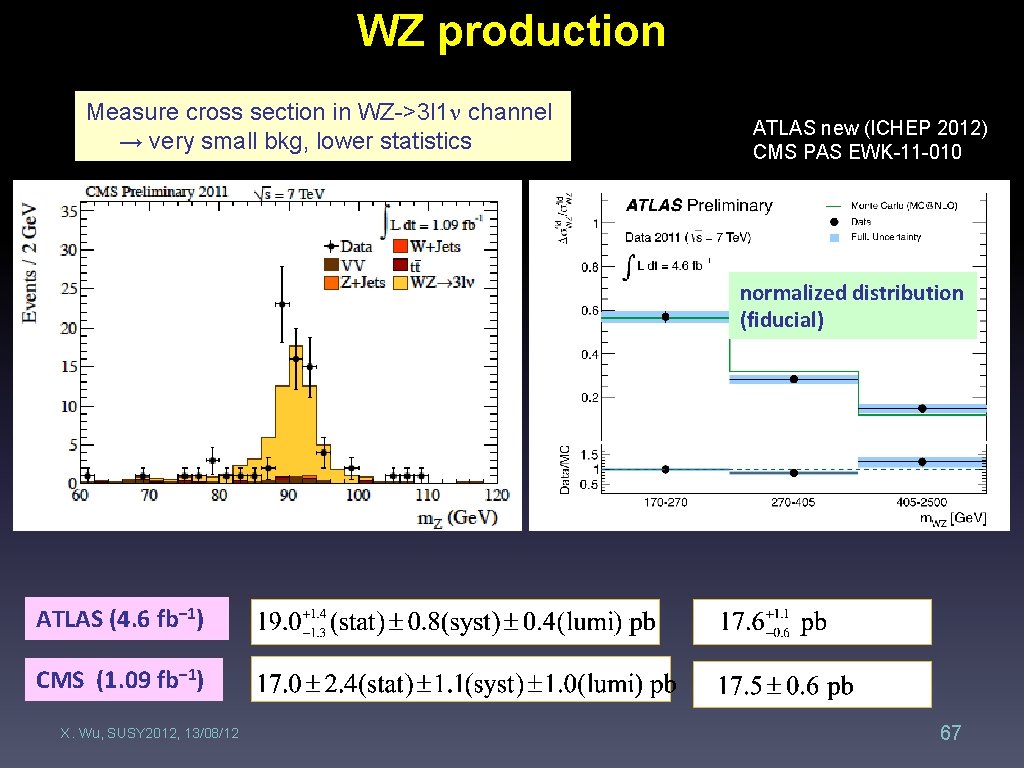
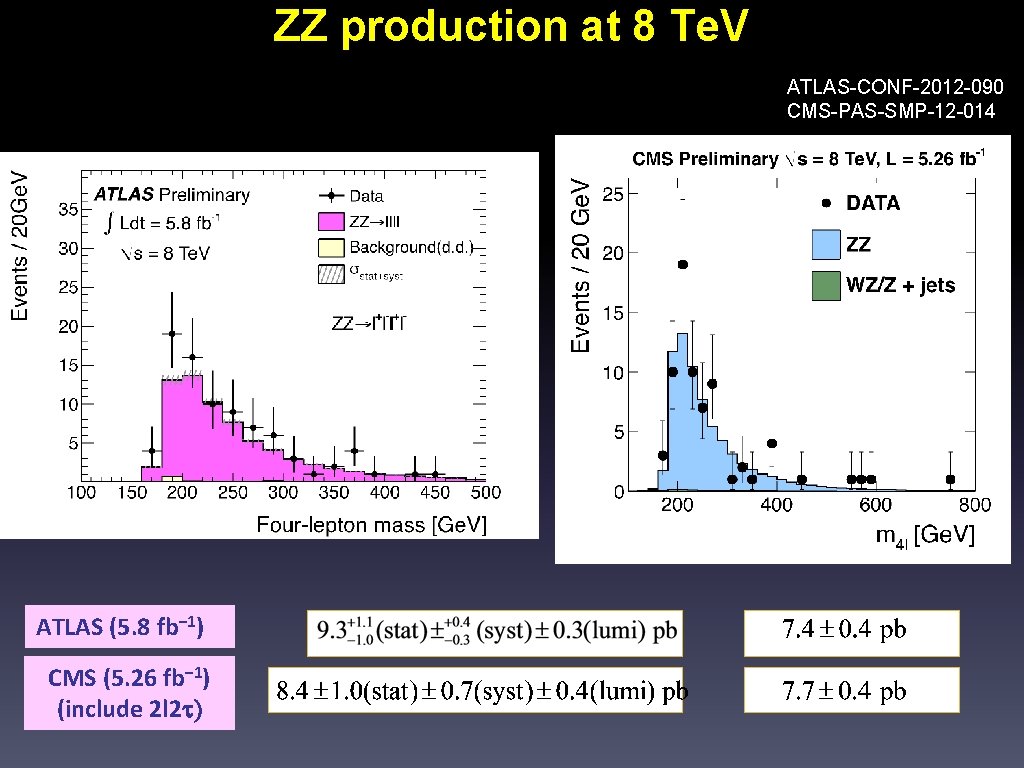
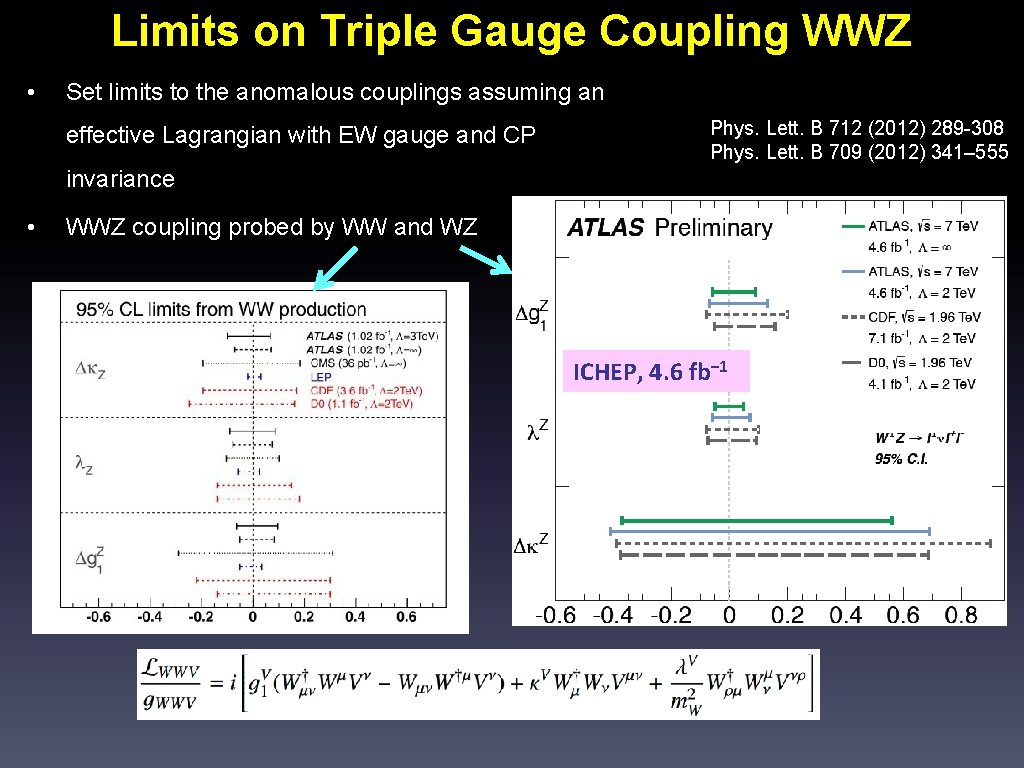
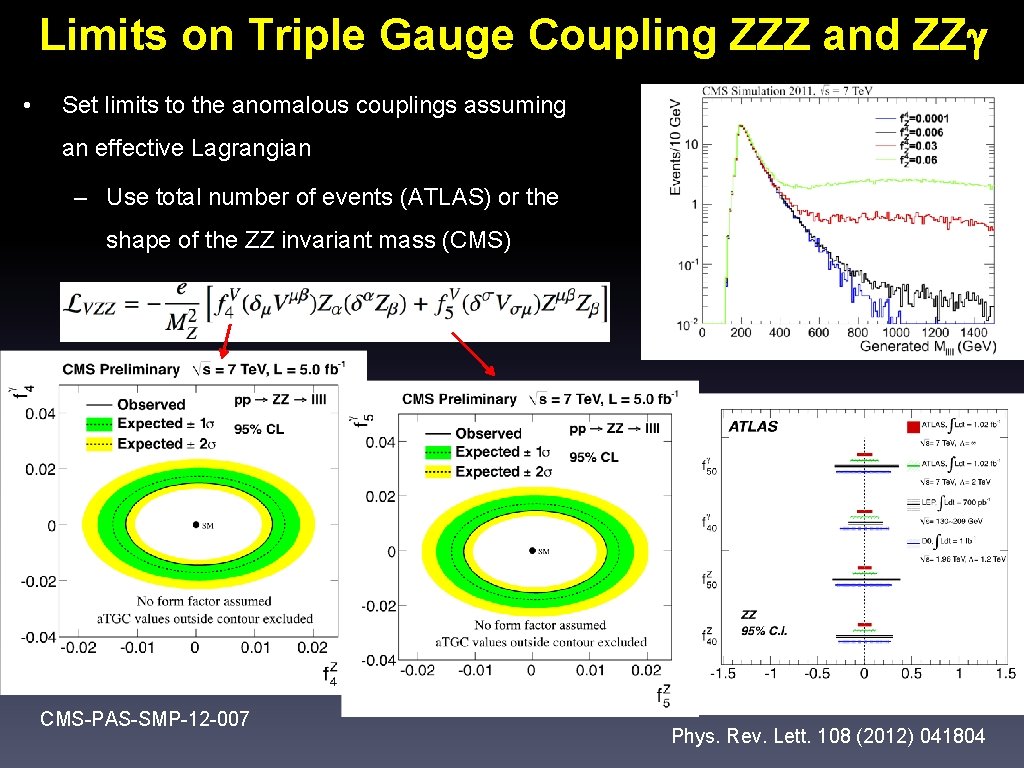
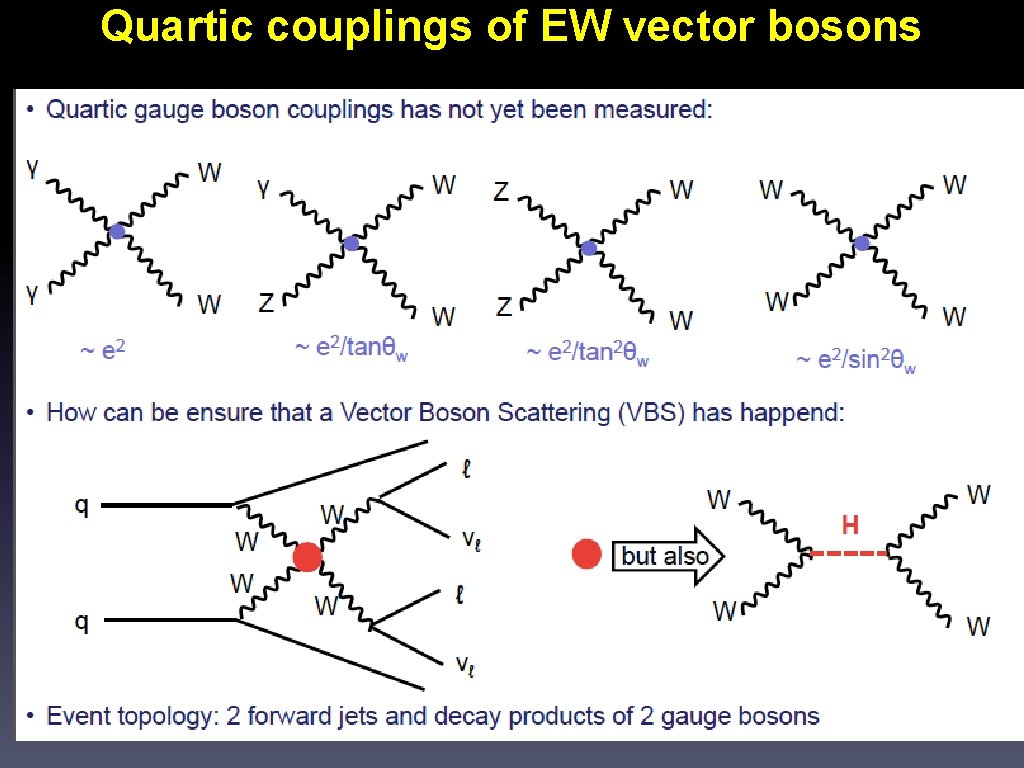
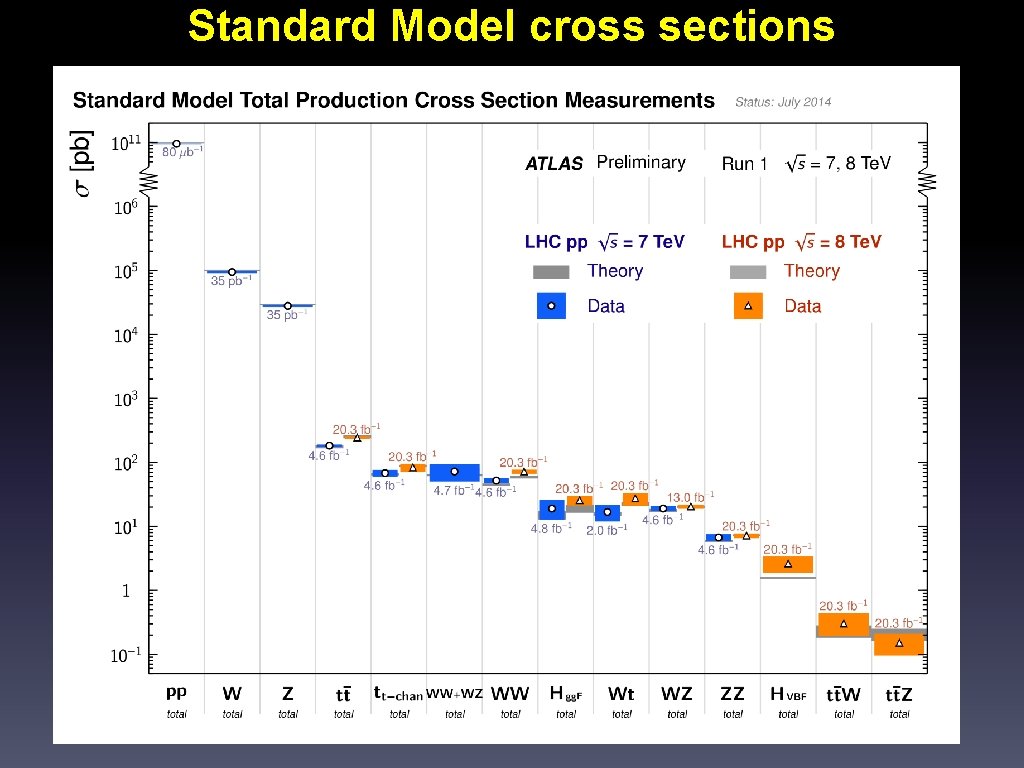
- Slides: 72
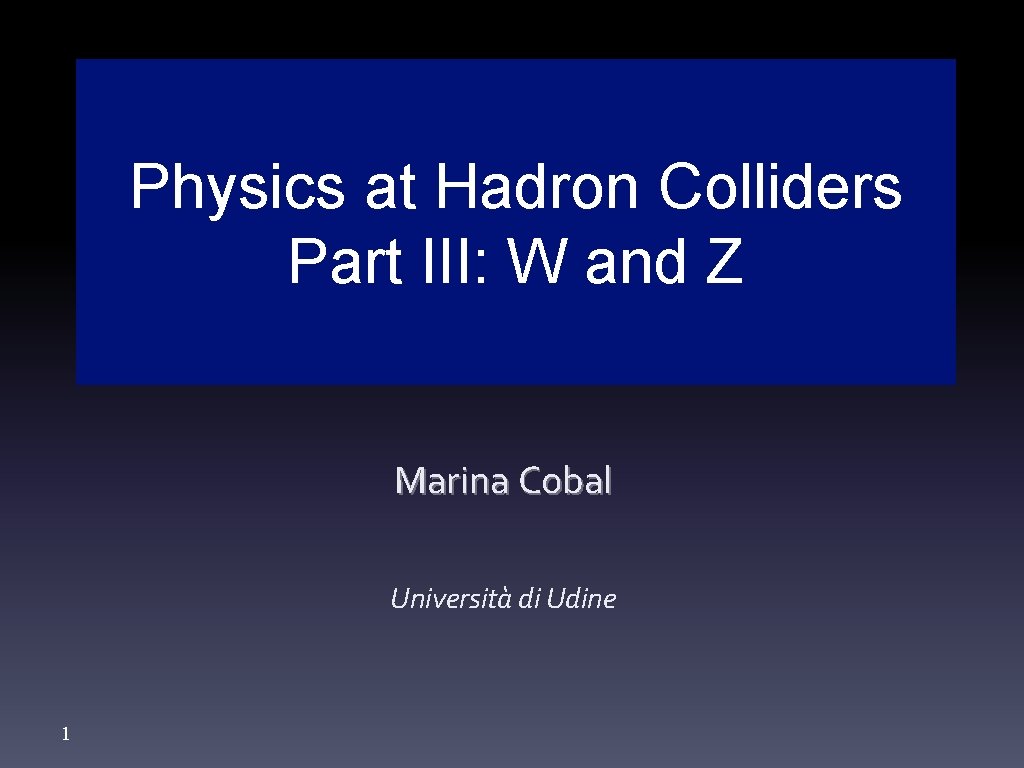
Physics at Hadron Colliders Physics Tevatron: Partat. III: the W and Z Lecture I Marina Cobal Università di Udine 1
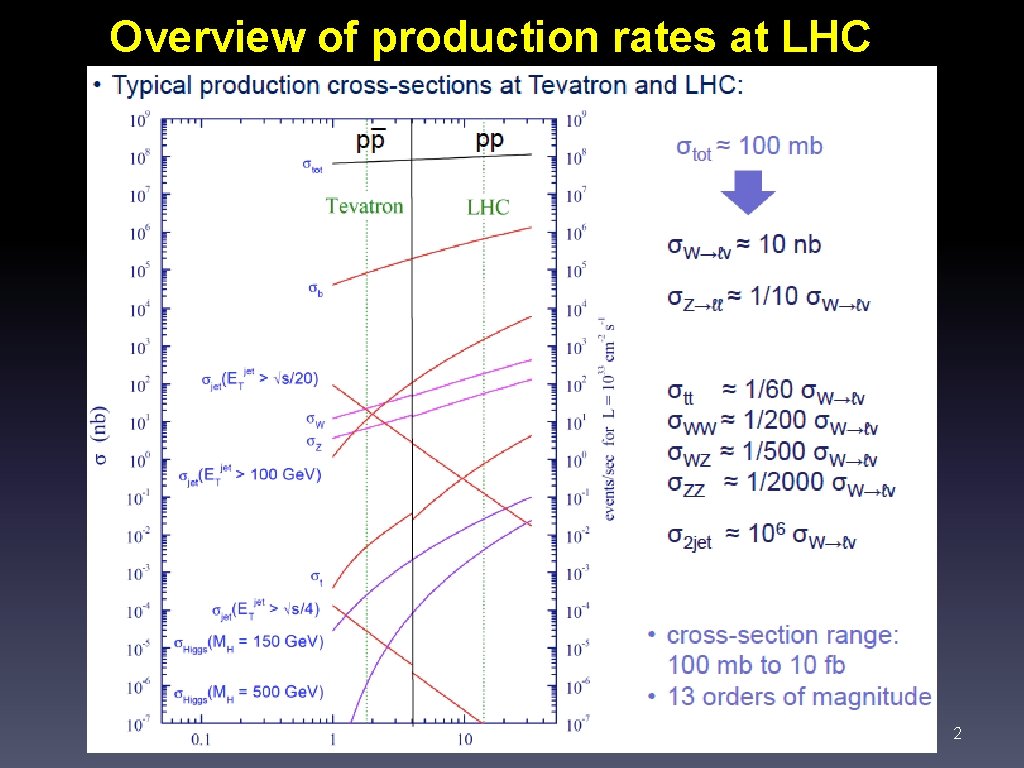
Overview of production rates at LHC 2
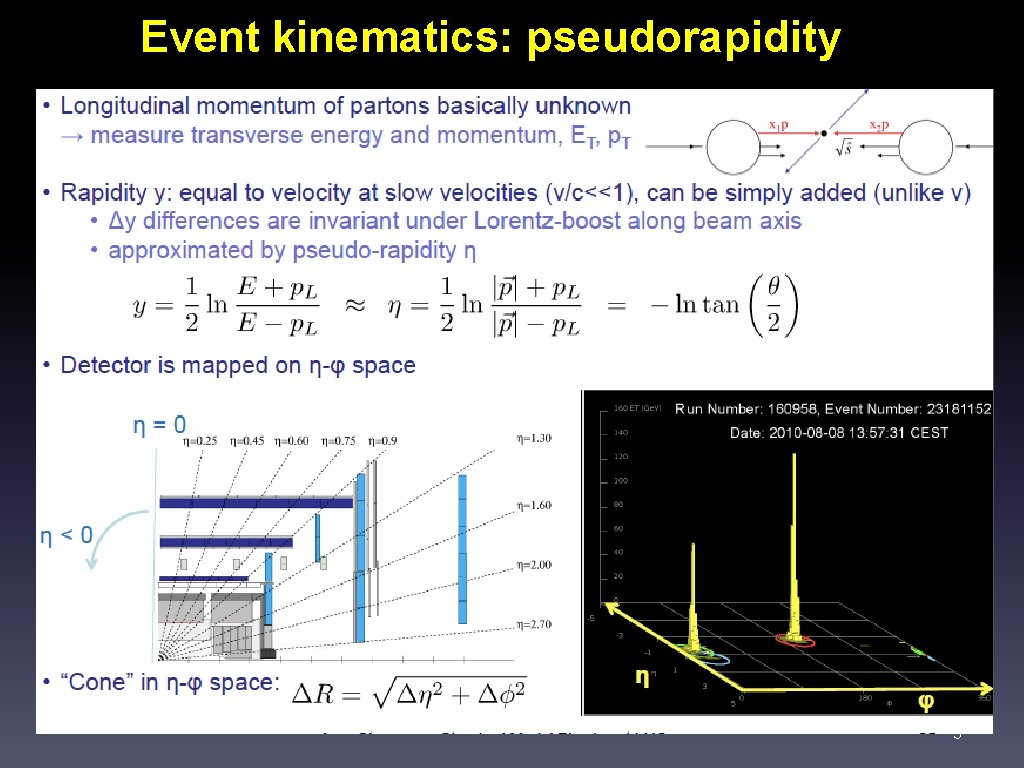
Event kinematics: pseudorapidity 3
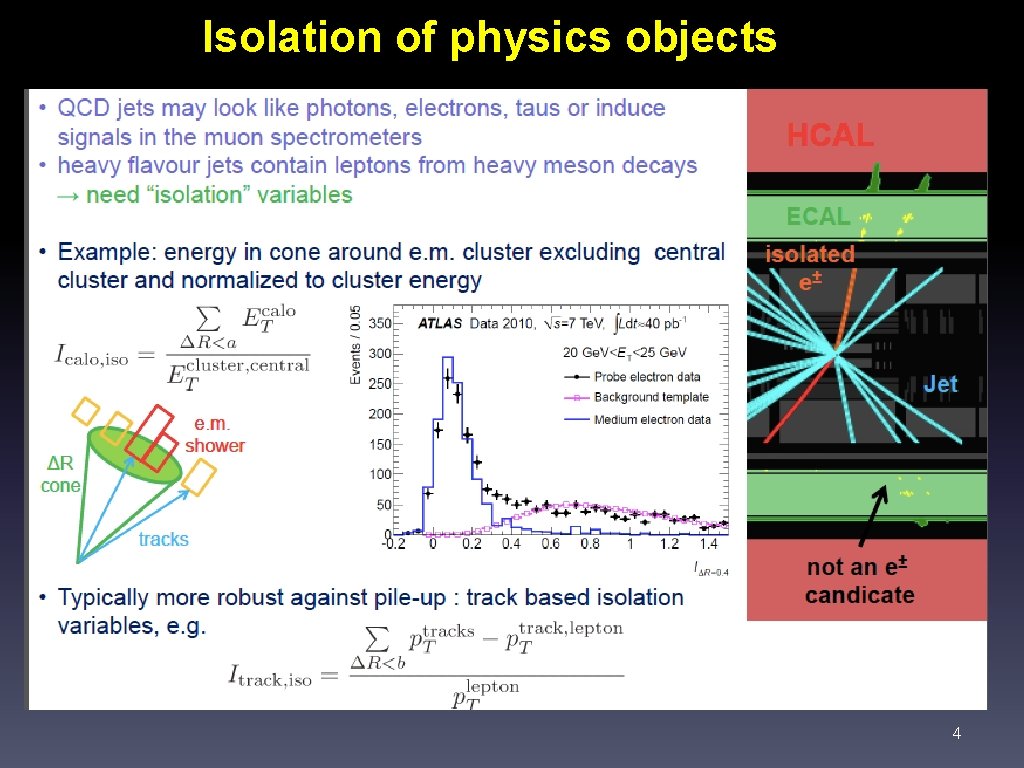
Isolation of physics objects 4
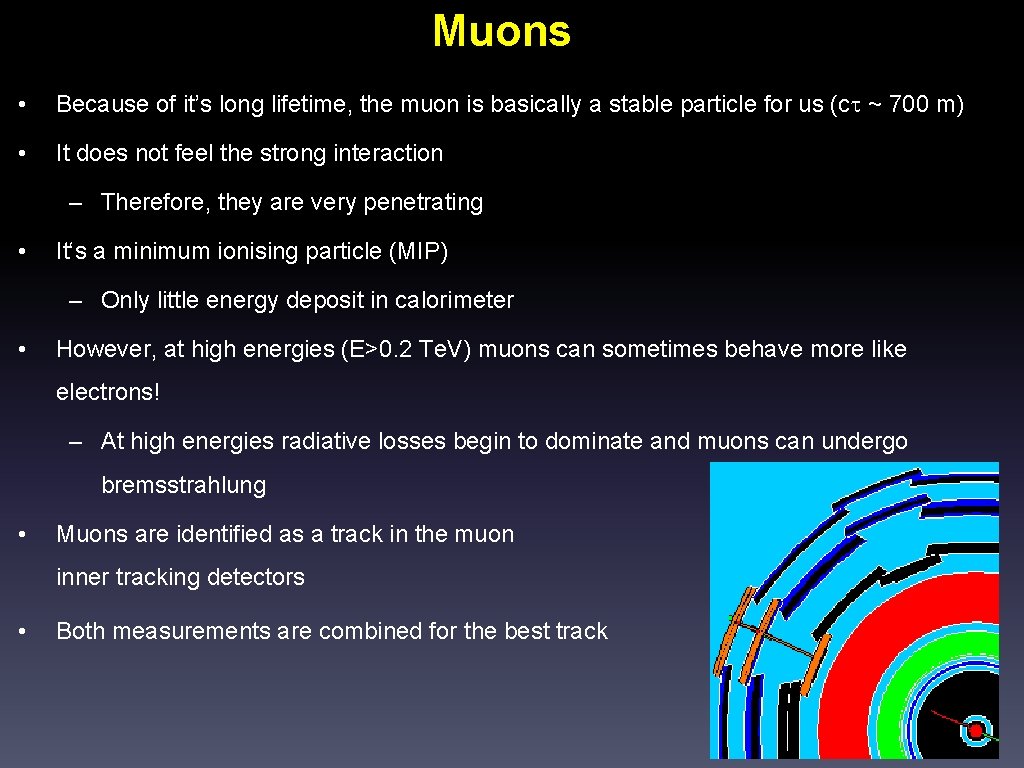
Muons • Because of it’s long lifetime, the muon is basically a stable particle for us (c ~ 700 m) • It does not feel the strong interaction – Therefore, they are very penetrating • It‘s a minimum ionising particle (MIP) – Only little energy deposit in calorimeter • However, at high energies (E>0. 2 Te. V) muons can sometimes behave more like electrons! – At high energies radiative losses begin to dominate and muons can undergo bremsstrahlung • Muons are identified as a track in the muon chambers and in the inner tracking detectors • Both measurements are combined for the best track results 5
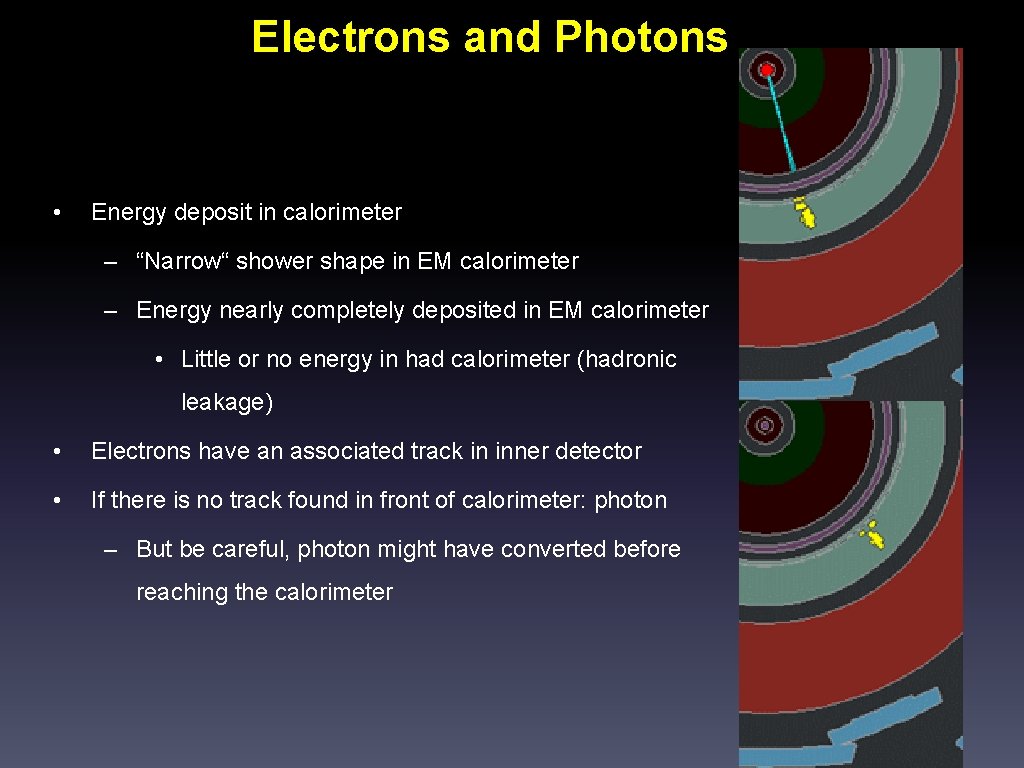
Electrons and Photons • Energy deposit in calorimeter – “Narrow“ shower shape in EM calorimeter – Energy nearly completely deposited in EM calorimeter • Little or no energy in had calorimeter (hadronic leakage) • Electrons have an associated track in inner detector • If there is no track found in front of calorimeter: photon – But be careful, photon might have converted before reaching the calorimeter 6
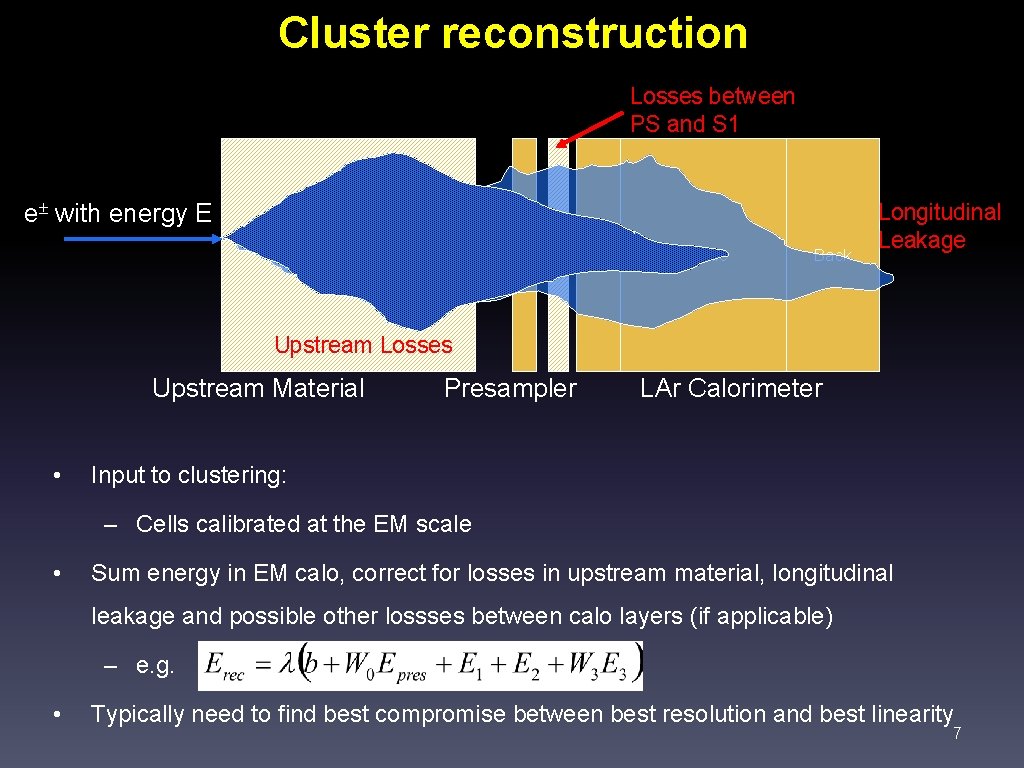
Cluster reconstruction Losses between PS and S 1 e with energy E strips Middle Back Longitudinal Leakage Upstream Losses Upstream Material • Presampler LAr Calorimeter Input to clustering: – Cells calibrated at the EM scale • Sum energy in EM calo, correct for losses in upstream material, longitudinal leakage and possible other lossses between calo layers (if applicable) – e. g. • Typically need to find best compromise between best resolution and best linearity 7
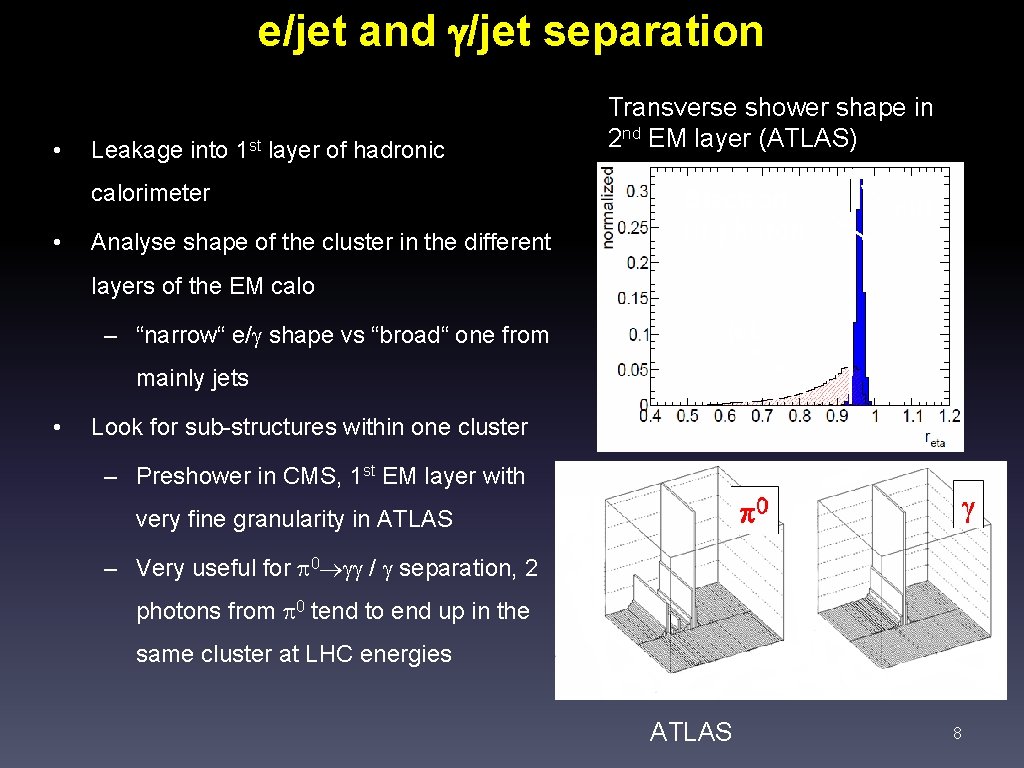
e/jet and /jet separation • Leakage into 1 st layer of hadronic calorimeter • Analyse shape of the cluster in the different Transverse shower shape in 2 nd EM layer (ATLAS) Electron or photon cut layers of the EM calo – “narrow“ e/ shape vs “broad“ one from jet mainly jets • Look for sub-structures within one cluster – Preshower in CMS, 1 st EM layer with very fine granularity in ATLAS – Very useful for 0 / separation, 2 photons from 0 tend to end up in the same cluster at LHC energies ATLAS 8
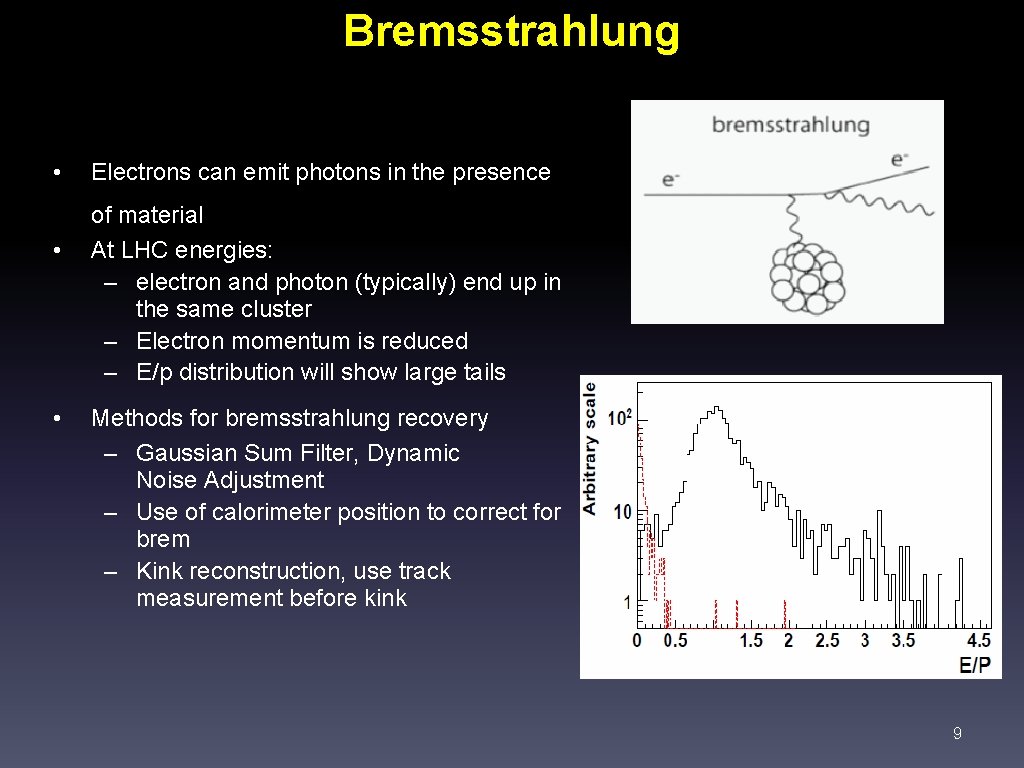
Bremsstrahlung • • • Electrons can emit photons in the presence of material At LHC energies: – electron and photon (typically) end up in the same cluster – Electron momentum is reduced – E/p distribution will show large tails Methods for bremsstrahlung recovery – Gaussian Sum Filter, Dynamic Noise Adjustment – Use of calorimeter position to correct for brem – Kink reconstruction, use track measurement before kink 9
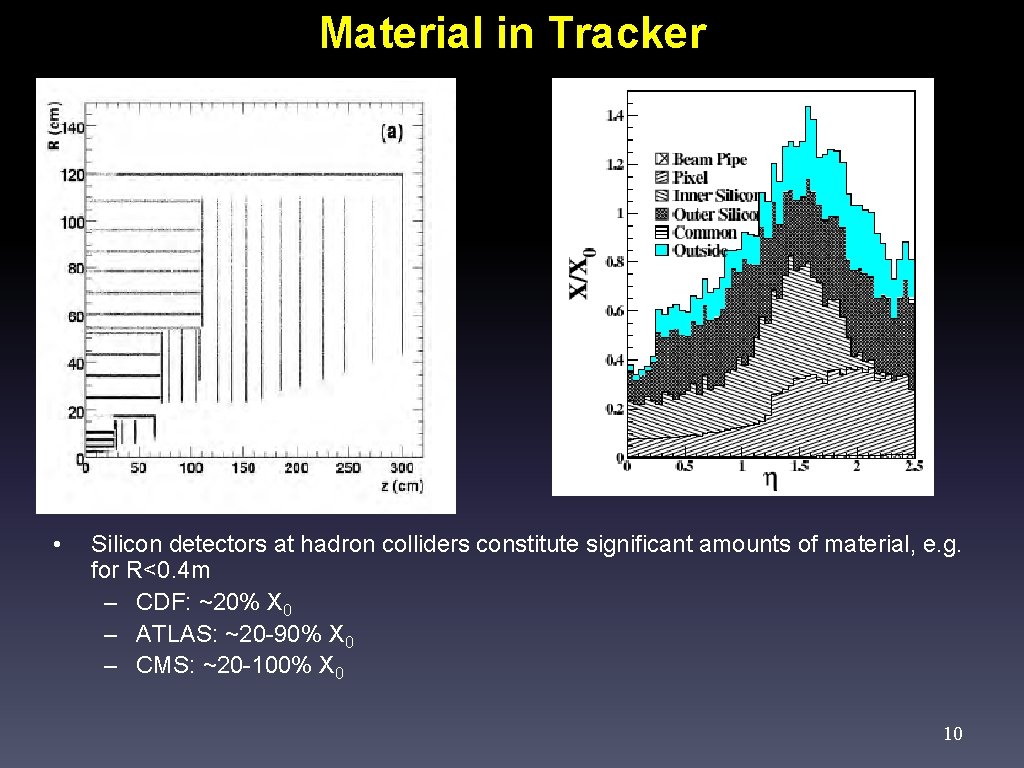
Material in Tracker CMS • CMS Silicon detectors at hadron colliders constitute significant amounts of material, e. g. for R<0. 4 m – CDF: ~20% X 0 – ATLAS: ~20 -90% X 0 – CMS: ~20 -100% X 0 10
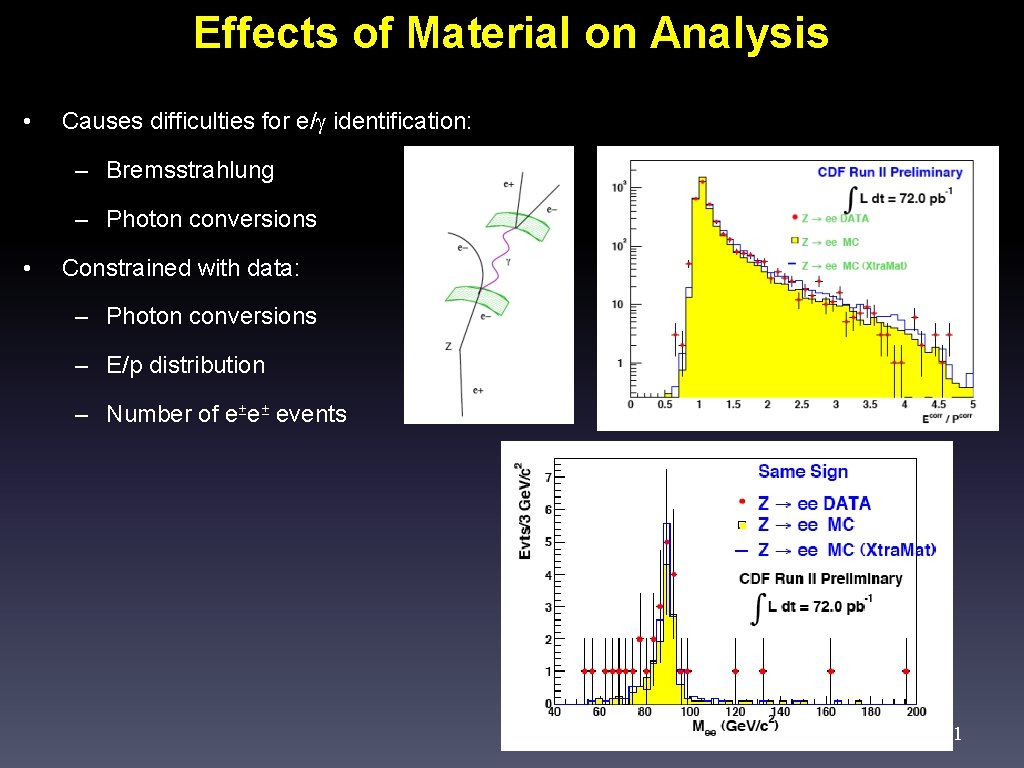
Effects of Material on Analysis • Causes difficulties for e/ identification: – Bremsstrahlung – Photon conversions • Constrained with data: – Photon conversions – E/p distribution – Number of e±e± events 11
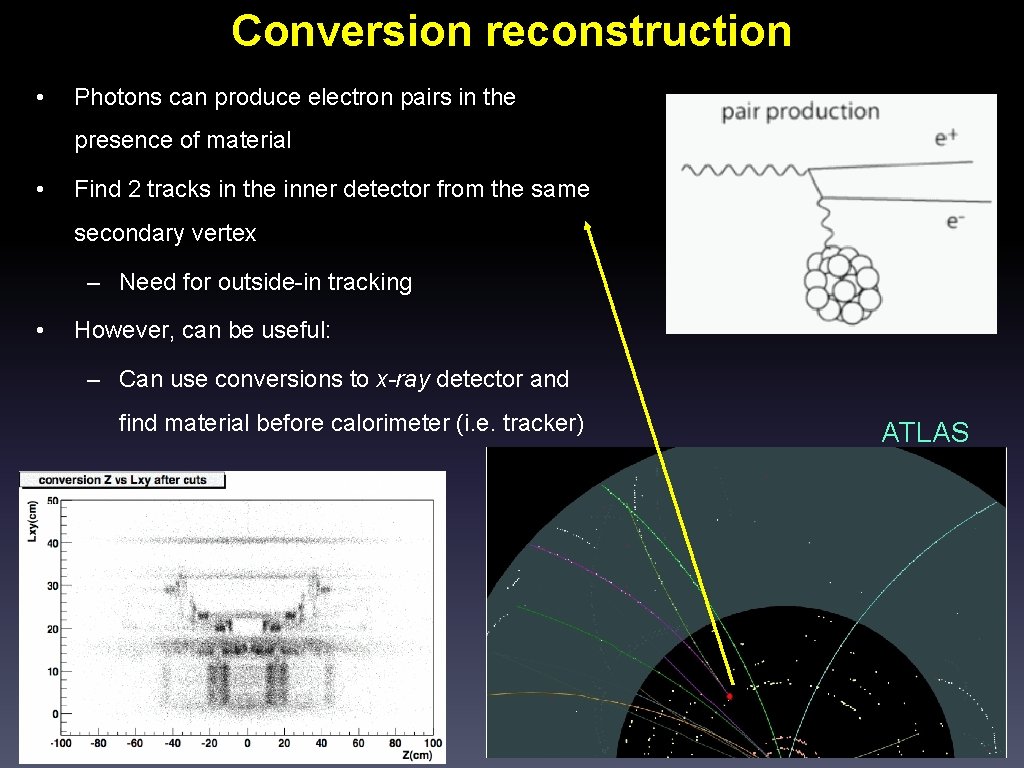
Conversion reconstruction • Photons can produce electron pairs in the presence of material • Find 2 tracks in the inner detector from the same secondary vertex – Need for outside-in tracking • However, can be useful: – Can use conversions to x-ray detector and find material before calorimeter (i. e. tracker) ATLAS CDF 12
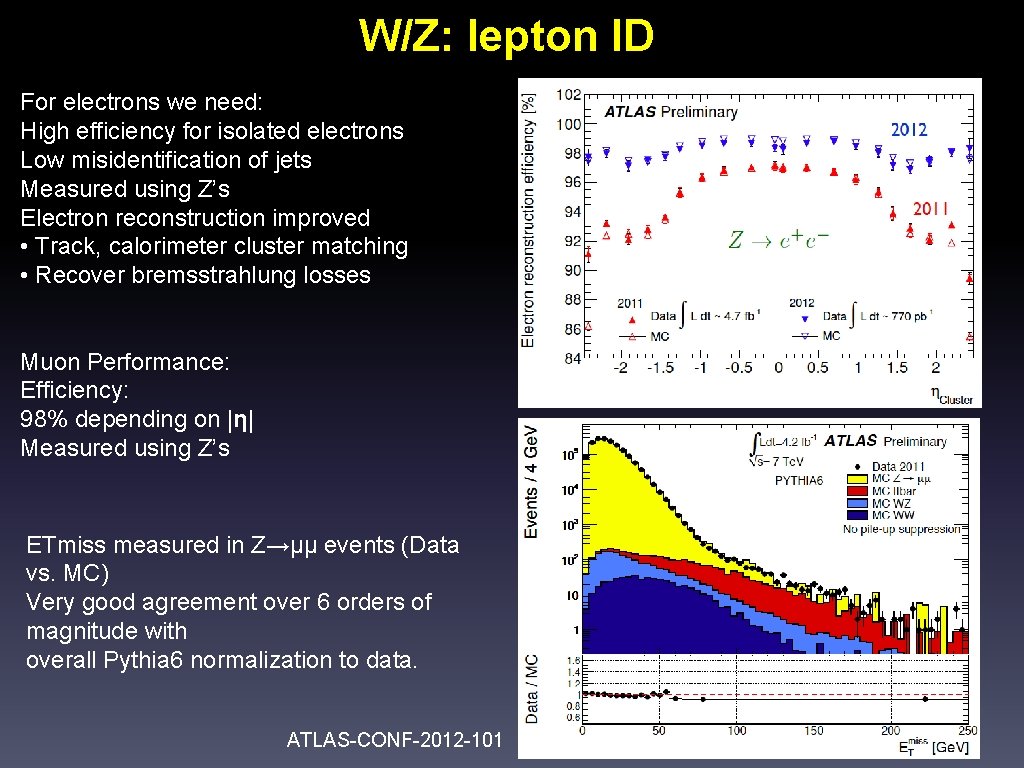
W/Z: lepton ID For electrons we need: High efficiency for isolated electrons Low misidentification of jets Measured using Z’s Electron reconstruction improved • Track, calorimeter cluster matching • Recover bremsstrahlung losses Muon Performance: Efficiency: 98% depending on |η| Measured using Z’s ETmiss measured in Z→μμ events (Data vs. MC) Very good agreement over 6 orders of magnitude with overall Pythia 6 normalization to data. ATLAS-CONF-2012 -101 13
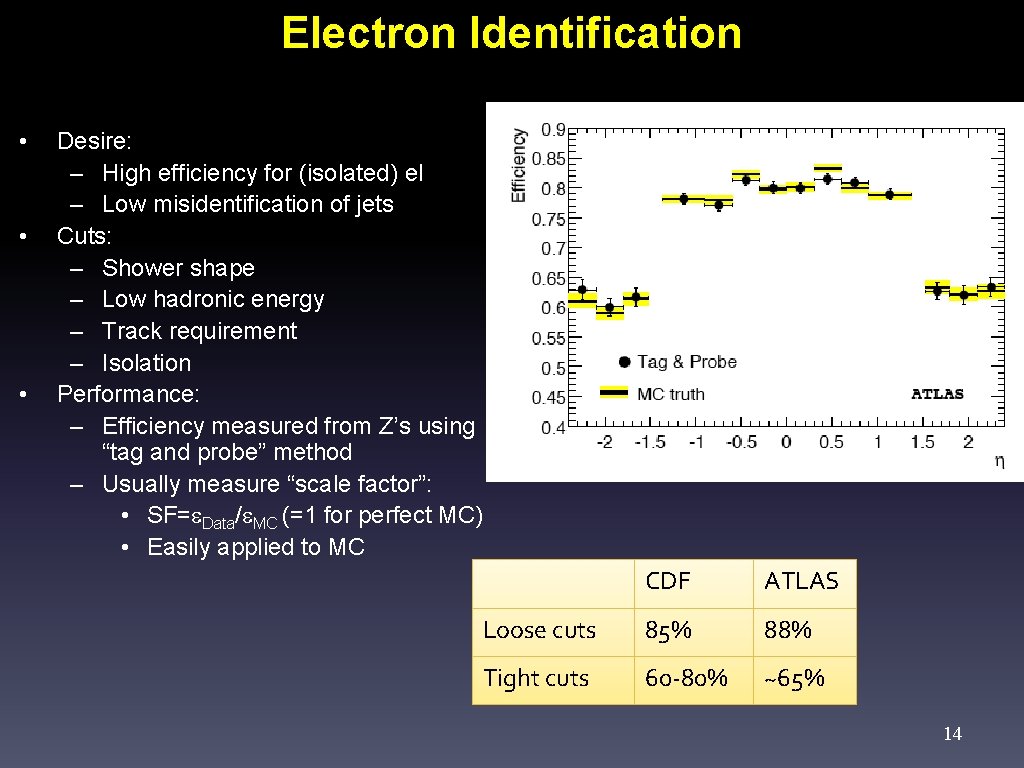
Electron Identification • • • Desire: – High efficiency for (isolated) el – Low misidentification of jets Cuts: – Shower shape – Low hadronic energy – Track requirement – Isolation Performance: – Efficiency measured from Z’s using “tag and probe” method – Usually measure “scale factor”: • SF= Data/ MC (=1 for perfect MC) • Easily applied to MC CDF ATLAS Loose cuts 85% 88% Tight cuts 60 -80% ~65% 14
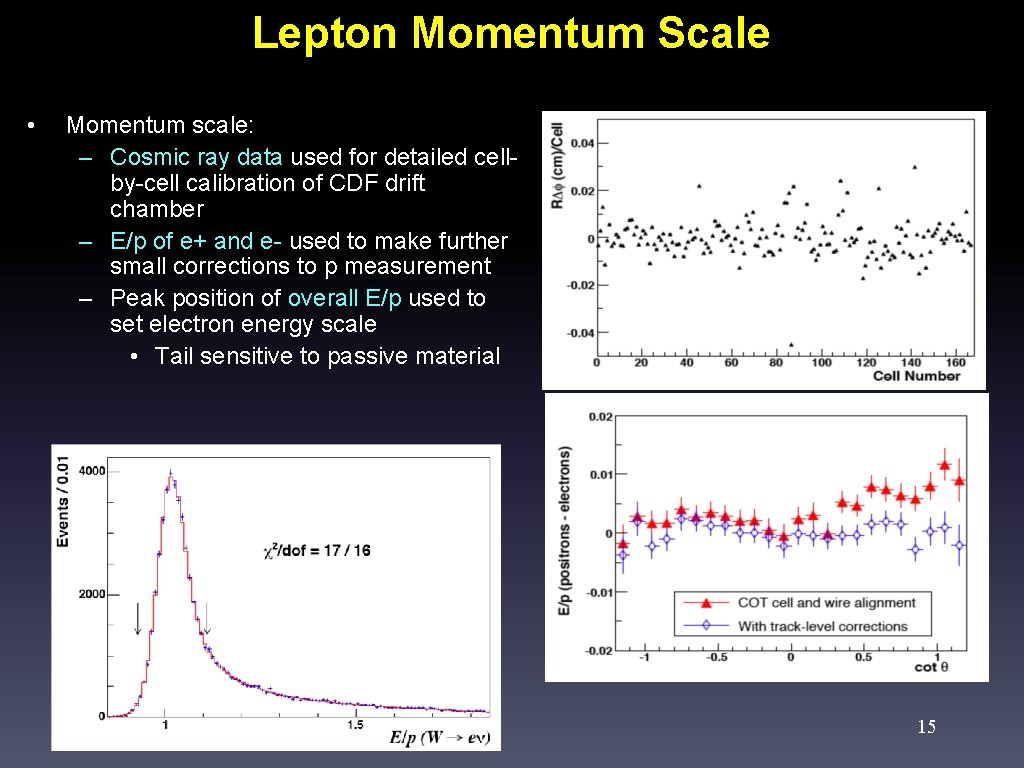
Lepton Momentum Scale • Momentum scale: – Cosmic ray data used for detailed cellby-cell calibration of CDF drift chamber – E/p of e+ and e- used to make further small corrections to p measurement – Peak position of overall E/p used to set electron energy scale • Tail sensitive to passive material 15
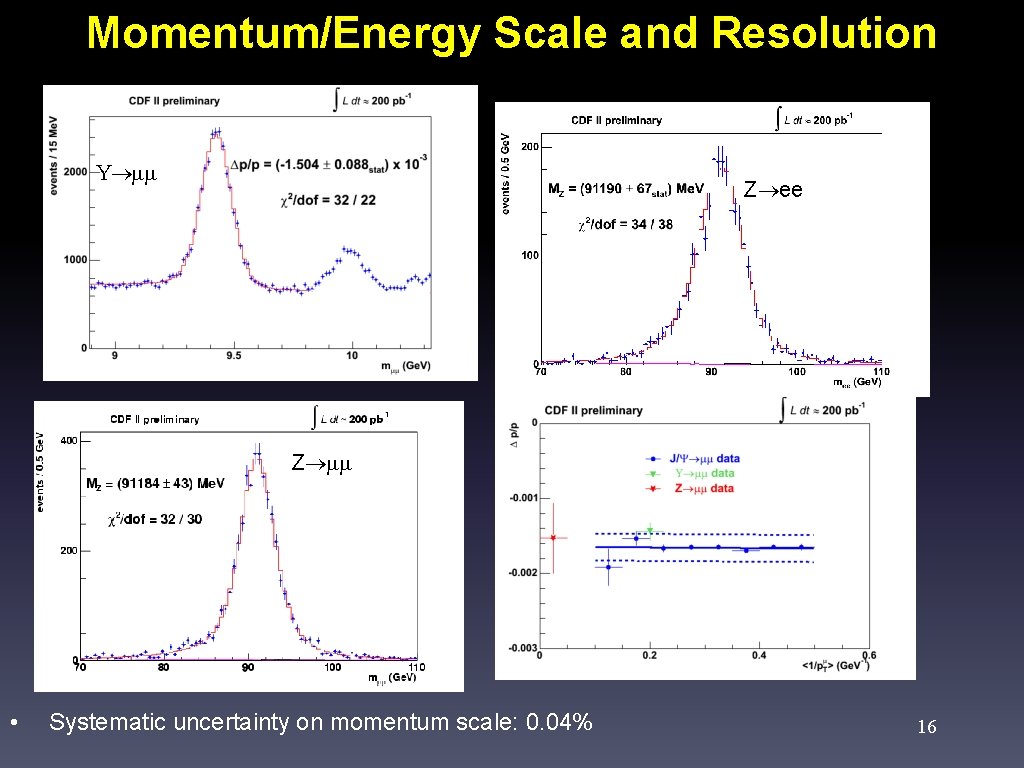
Momentum/Energy Scale and Resolution Z ee Z • Systematic uncertainty on momentum scale: 0. 04% 16
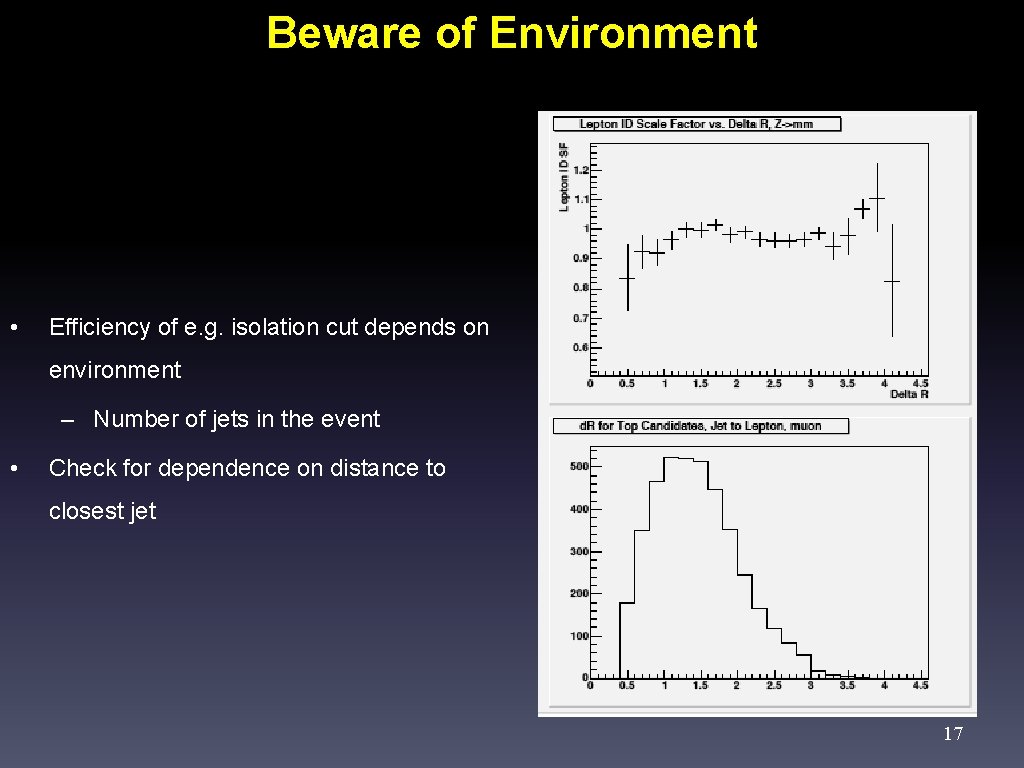
Beware of Environment • Efficiency of e. g. isolation cut depends on environment – Number of jets in the event • Check for dependence on distance to closest jet 17
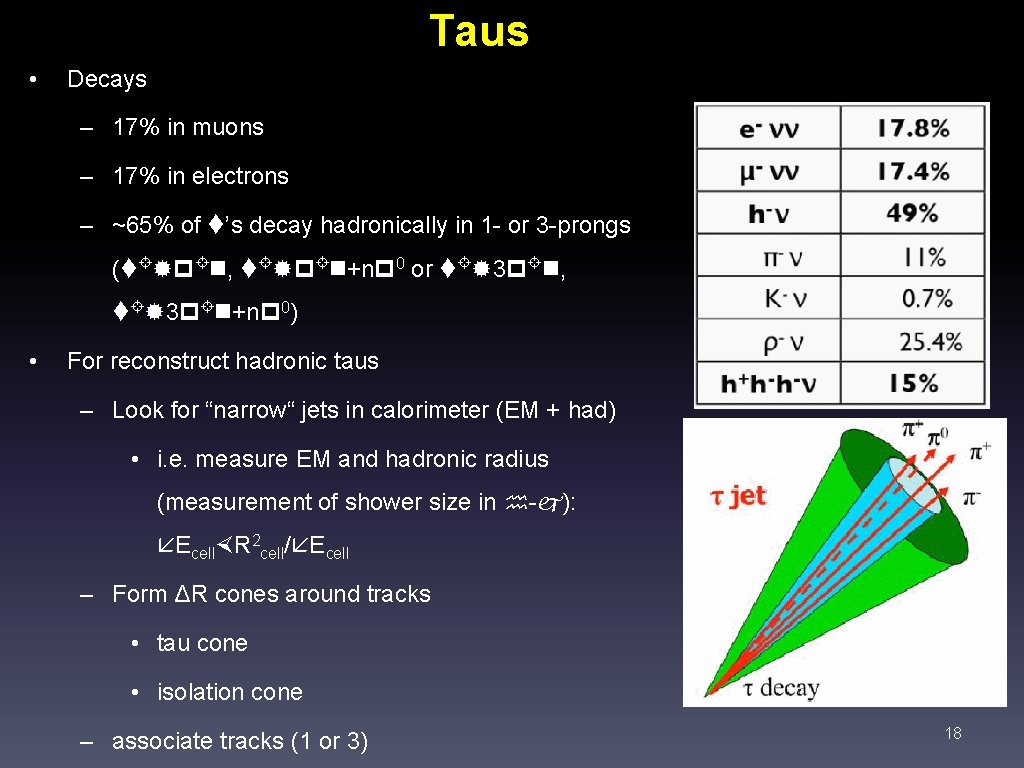
Taus • Decays – 17% in muons – 17% in electrons – ~65% of ’s decay hadronically in 1 - or 3 -prongs ( , +n 0 or 3 , 3 +n 0) • For reconstruct hadronic taus – Look for “narrow“ jets in calorimeter (EM + had) • i. e. measure EM and hadronic radius (measurement of shower size in - ): Ecell R 2 cell/ Ecell – Form ΔR cones around tracks • tau cone • isolation cone – associate tracks (1 or 3) 18
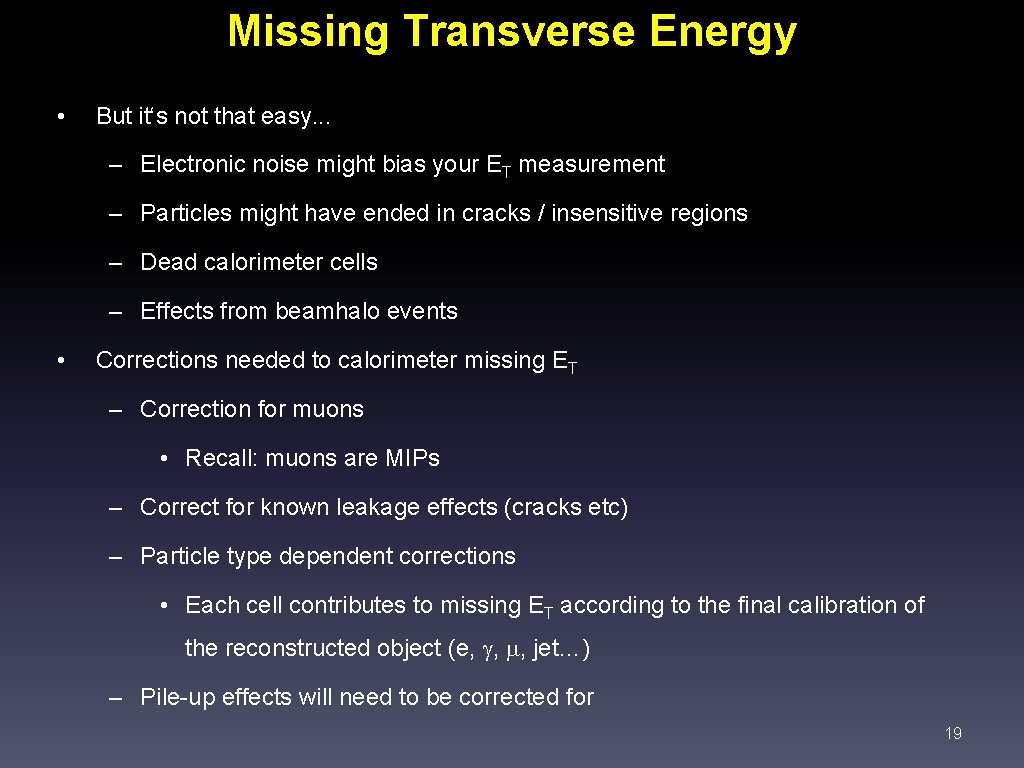
Missing Transverse Energy • But it‘s not that easy. . . – Electronic noise might bias your ET measurement – Particles might have ended in cracks / insensitive regions – Dead calorimeter cells – Effects from beamhalo events • Corrections needed to calorimeter missing ET – Correction for muons • Recall: muons are MIPs – Correct for known leakage effects (cracks etc) – Particle type dependent corrections • Each cell contributes to missing ET according to the final calibration of the reconstructed object (e, , , jet…) – Pile-up effects will need to be corrected for 19
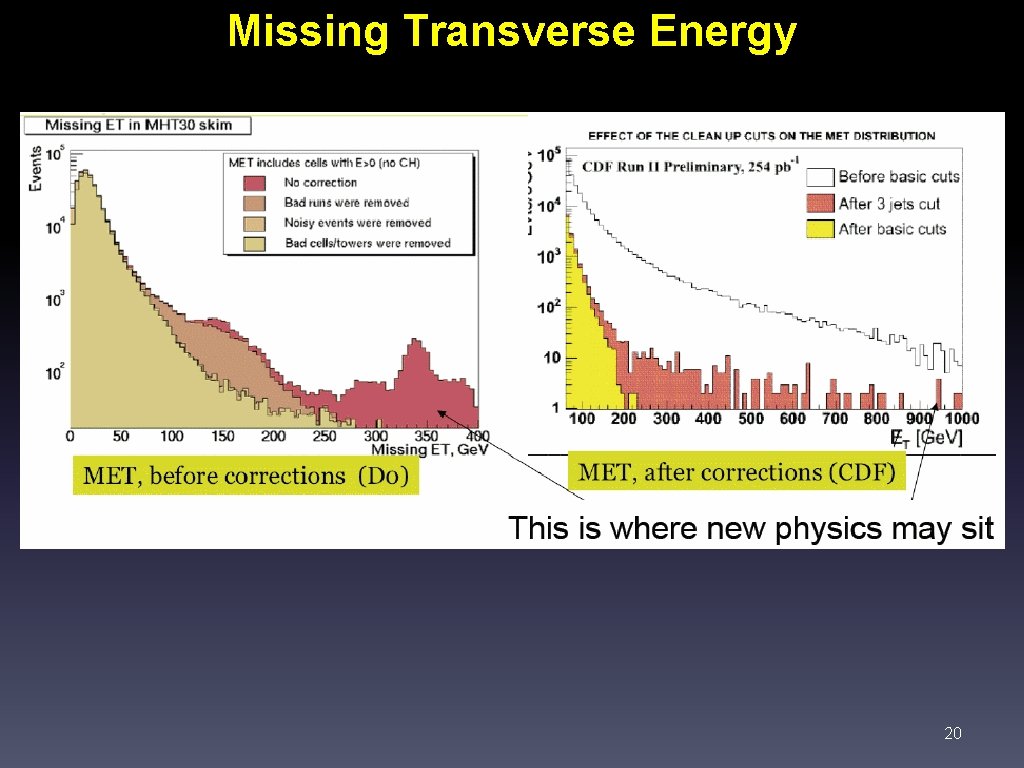
Missing Transverse Energy • Difficult to understand quantity 20
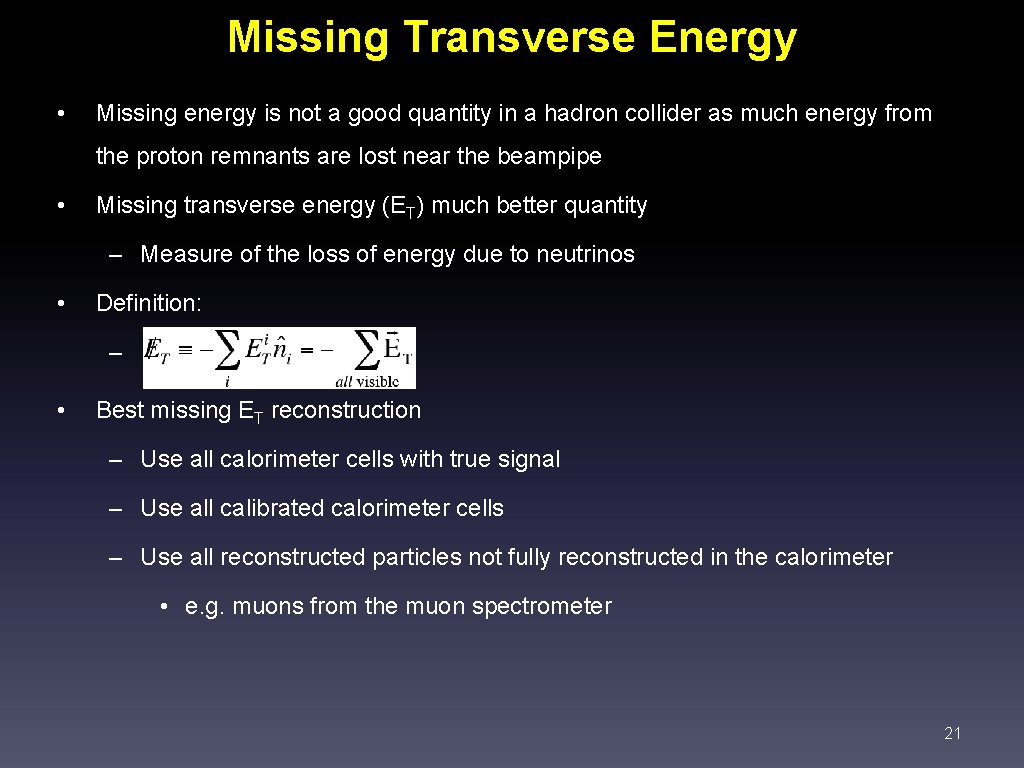
Missing Transverse Energy • Missing energy is not a good quantity in a hadron collider as much energy from the proton remnants are lost near the beampipe • Missing transverse energy (ET) much better quantity – Measure of the loss of energy due to neutrinos • Definition: – • Best missing ET reconstruction – Use all calorimeter cells with true signal – Use all calibrated calorimeter cells – Use all reconstructed particles not fully reconstructed in the calorimeter • e. g. muons from the muon spectrometer 21
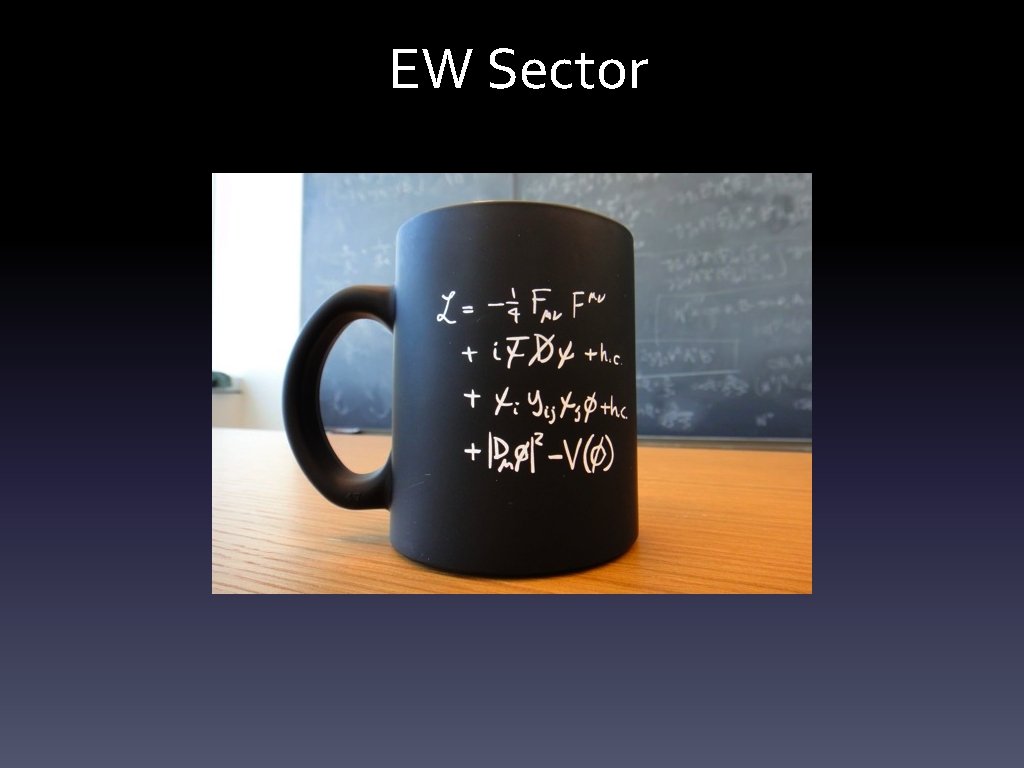
EW Sector
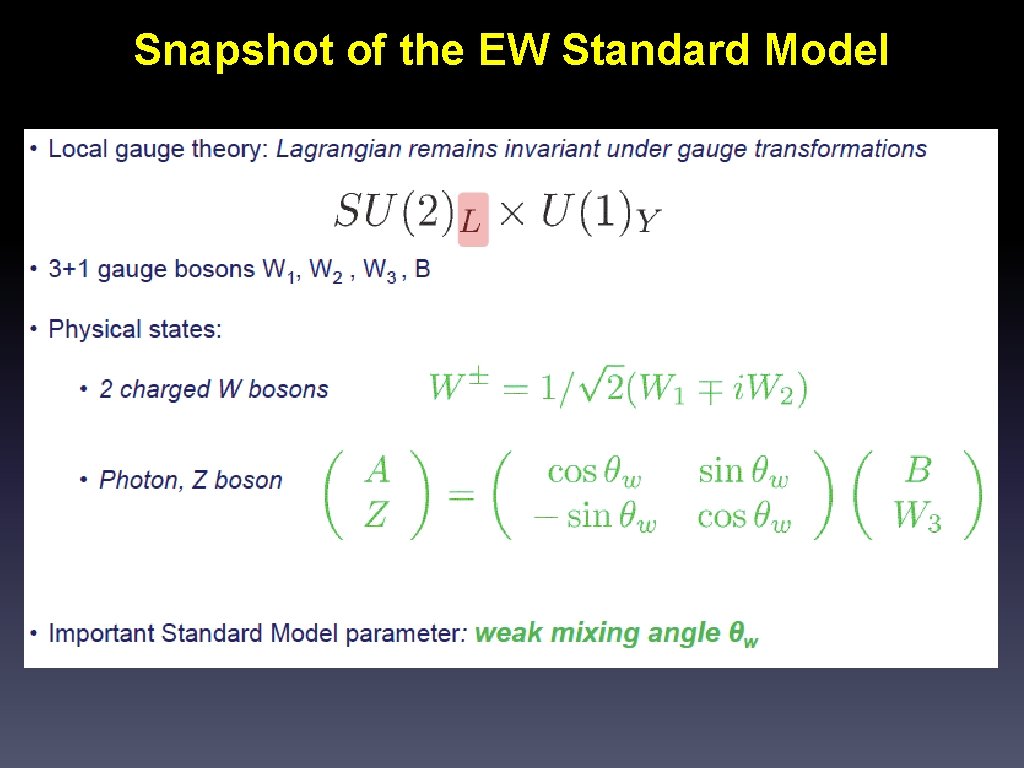
Snapshot of the EW Standard Model
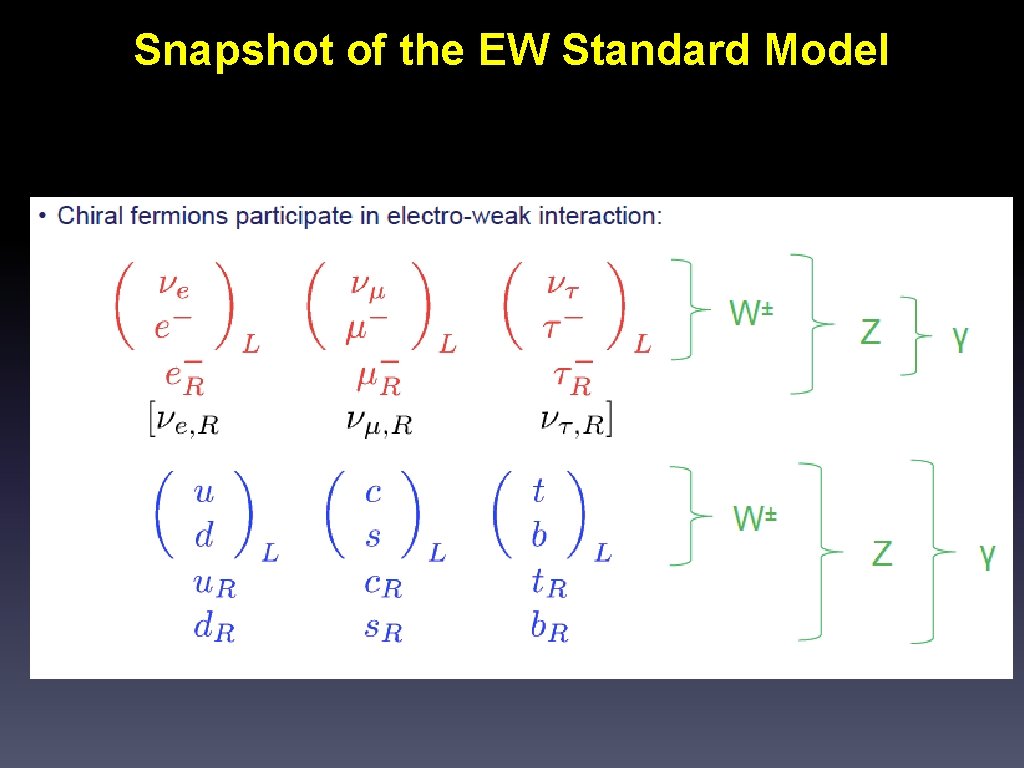
Snapshot of the EW Standard Model
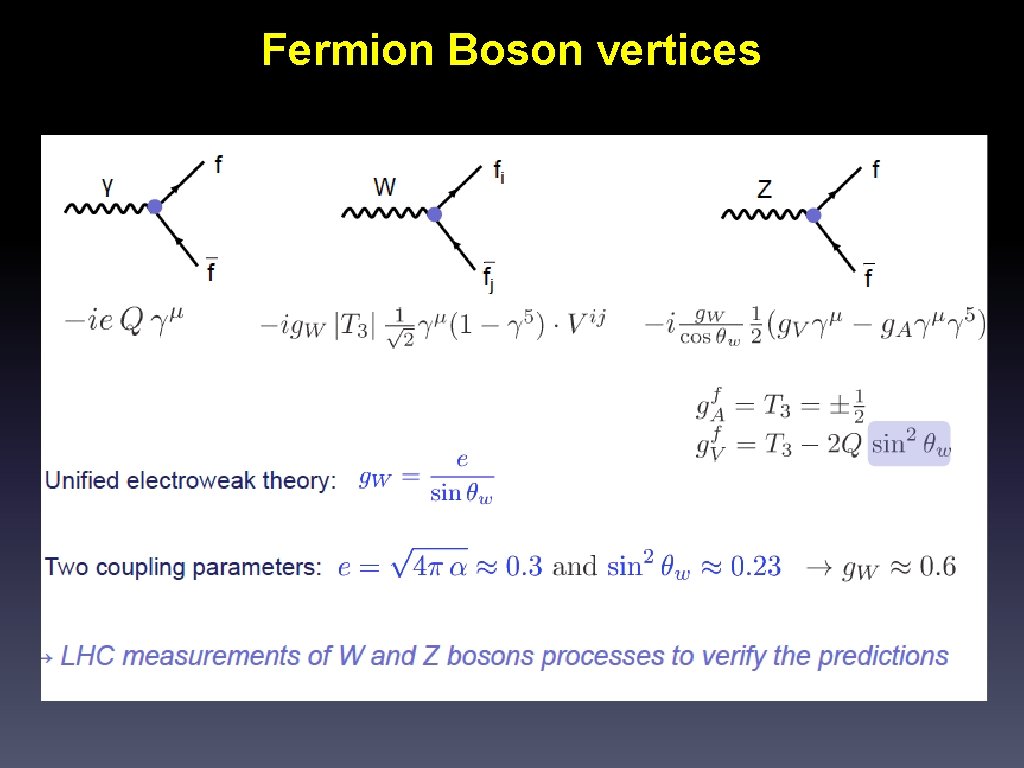
Fermion Boson vertices
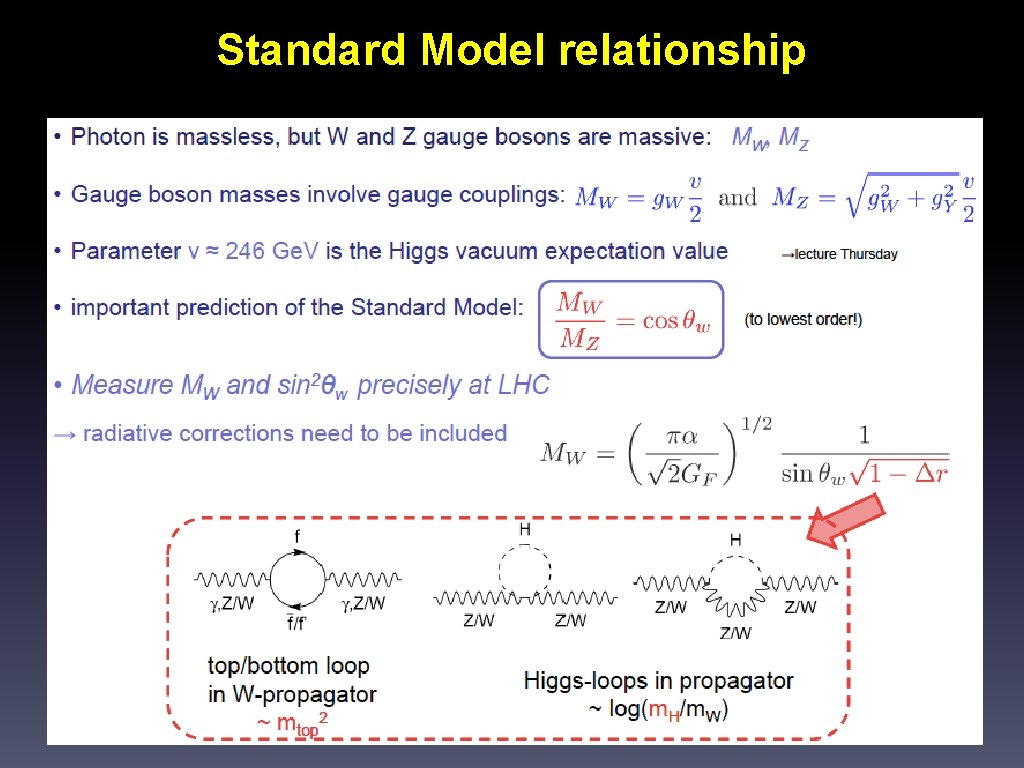
Standard Model relationship
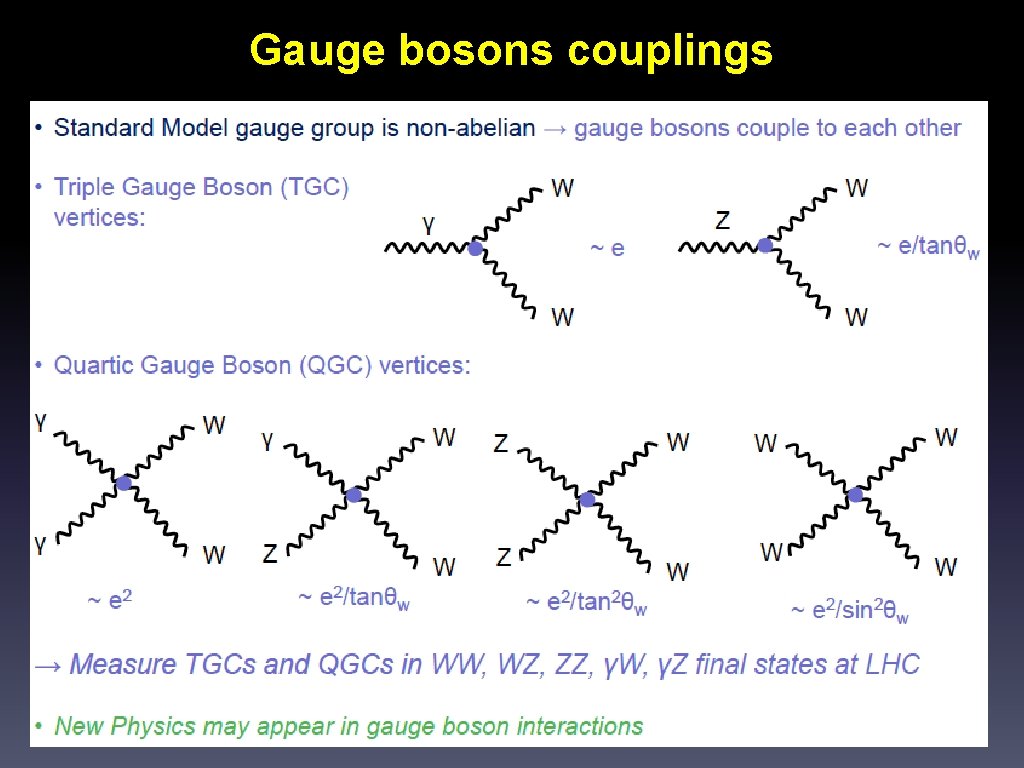
Gauge bosons couplings
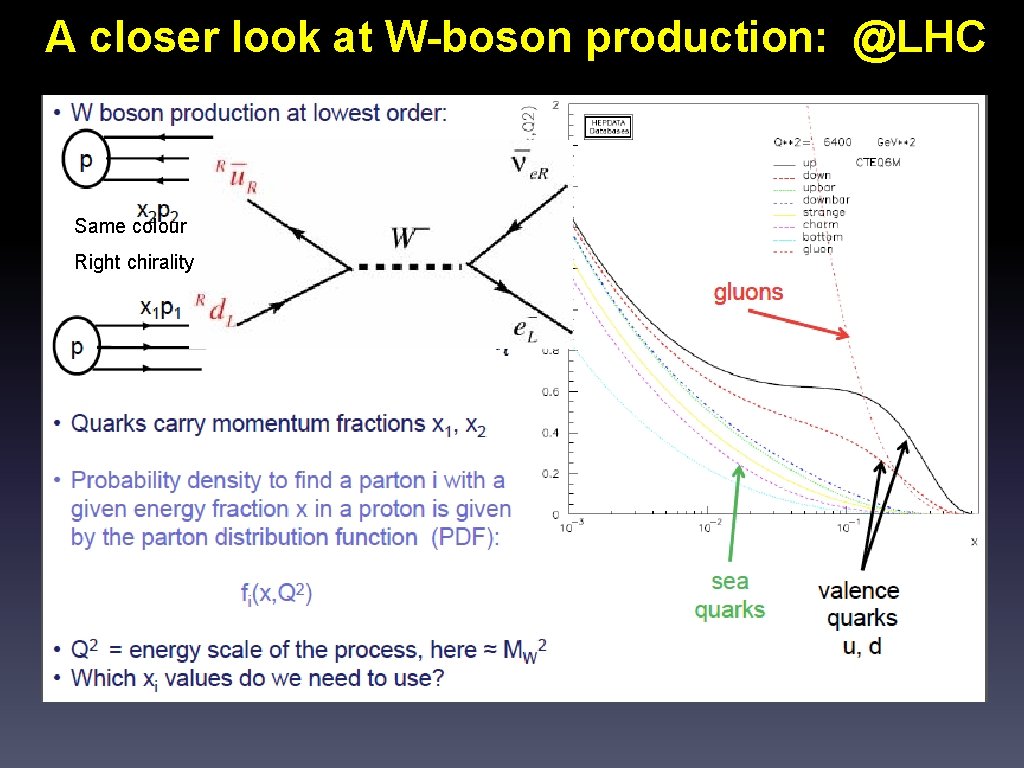
A closer look at W-boson production: @LHC Same colour Right chirality
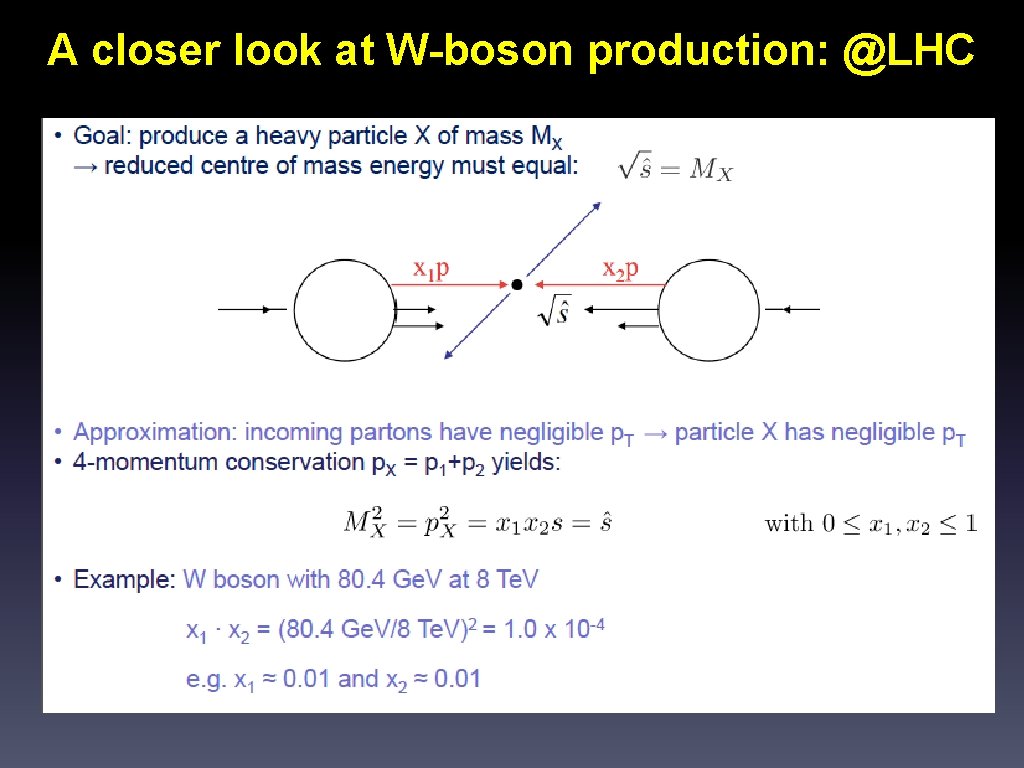
A closer look at W-boson production: @LHC
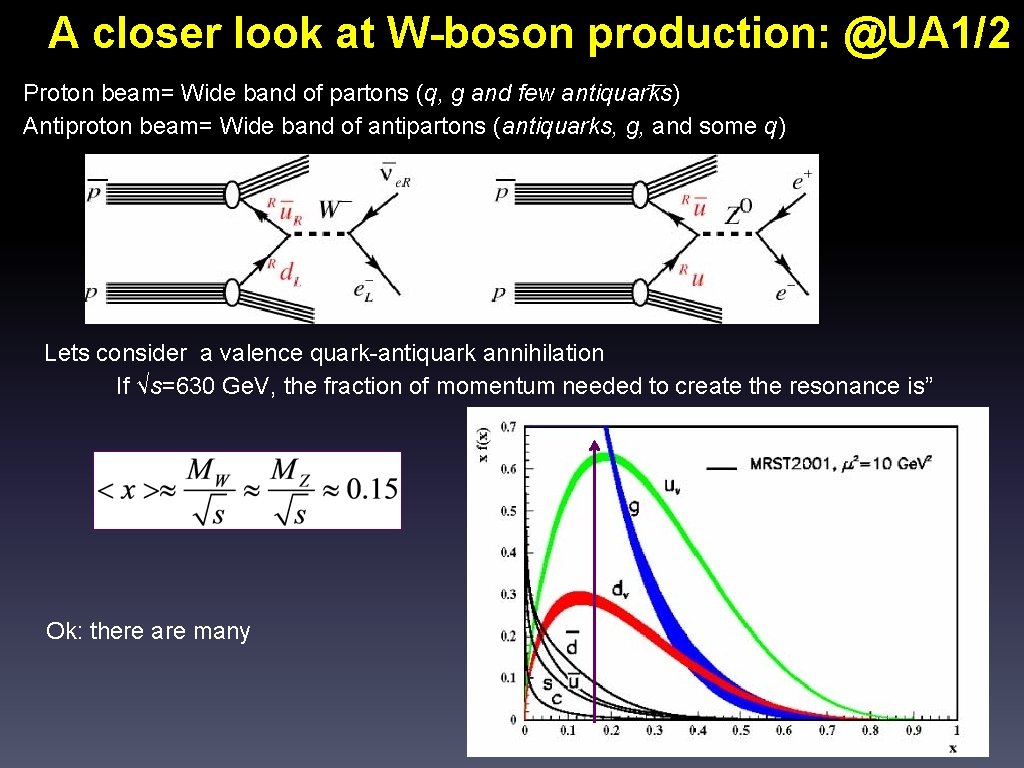
A closer look at W-boson production: @UA 1/2 Proton beam= Wide band of partons (q, g and few antiquarks) Antiproton beam= Wide band of antipartons (antiquarks, g, and some q) Lets consider a valence quark-antiquark annihilation If √s=630 Ge. V, the fraction of momentum needed to create the resonance is” Ok: there are many
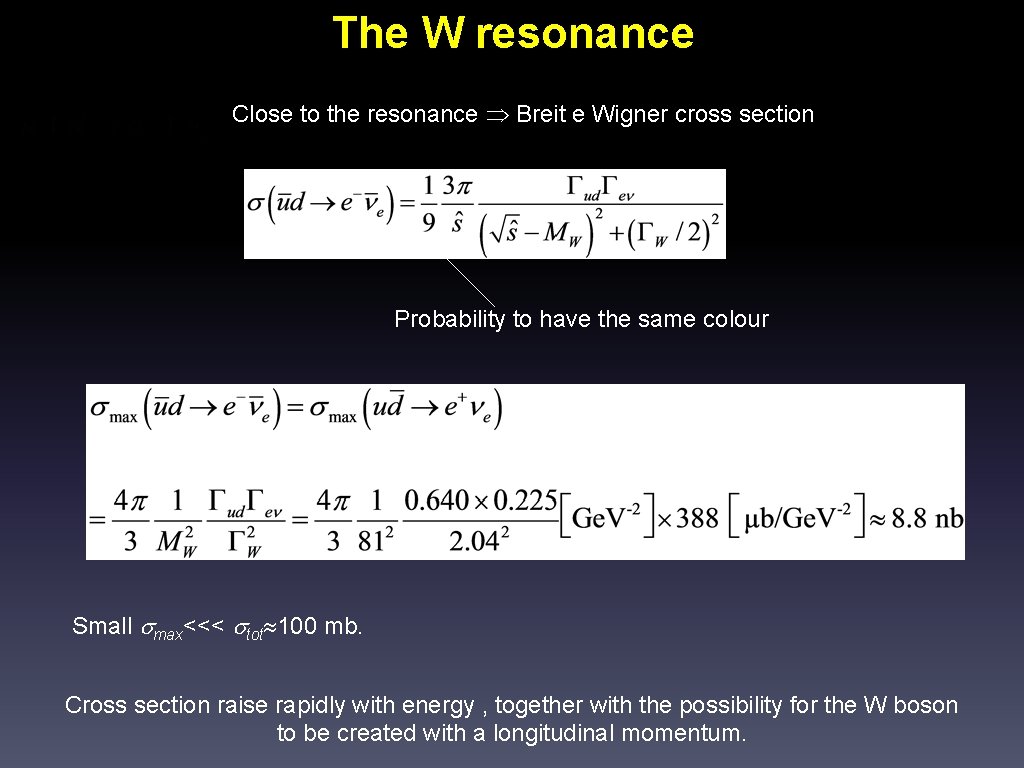
The W resonance Close to the resonance Breit e Wigner cross section Probability to have the same colour Small smax<<< stot 100 mb. Cross section raise rapidly with energy , together with the possibility for the W boson to be created with a longitudinal momentum.
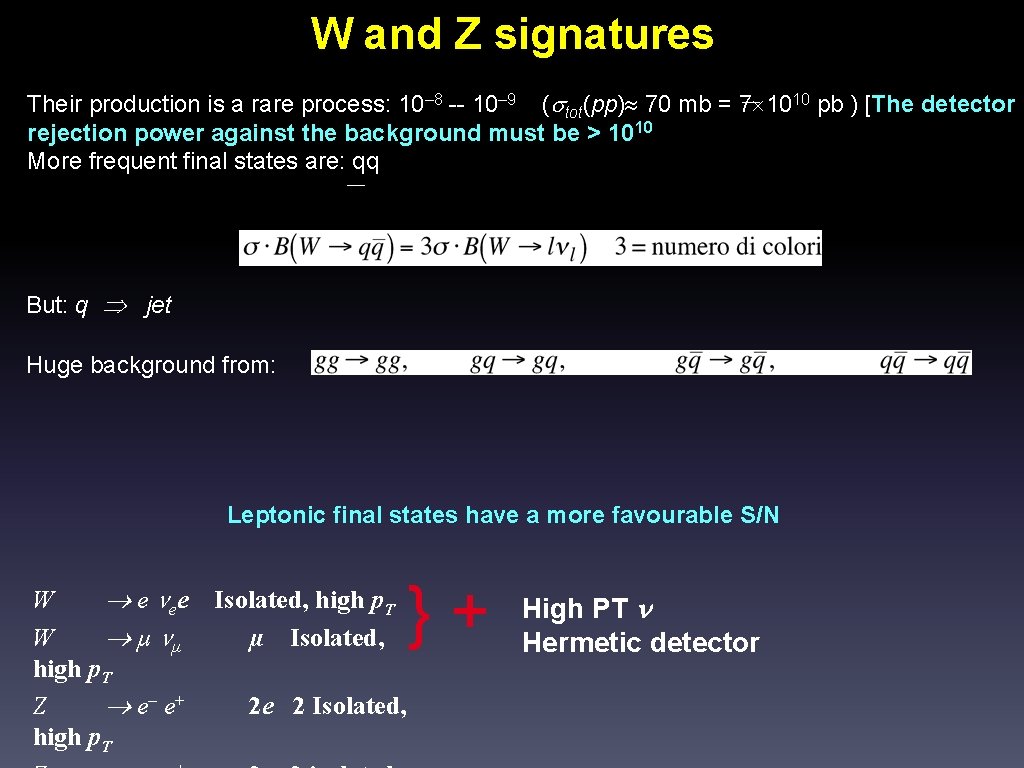
W and Z signatures Their production is a rare process: 10– 8 -- 10– 9 (stot(pp) 70 mb = 7 1010 pb ) [The detector rejection power against the background must be > 1010 More frequent final states are: qq But: q jet Huge background from: Leptonic final states have a more favourable S/N W e ne e W µ nµ high p. T Z e– e+ high p. T Isolated, high p. T µ Isolated, 2 e 2 Isolated, }+ High PT n Hermetic detector
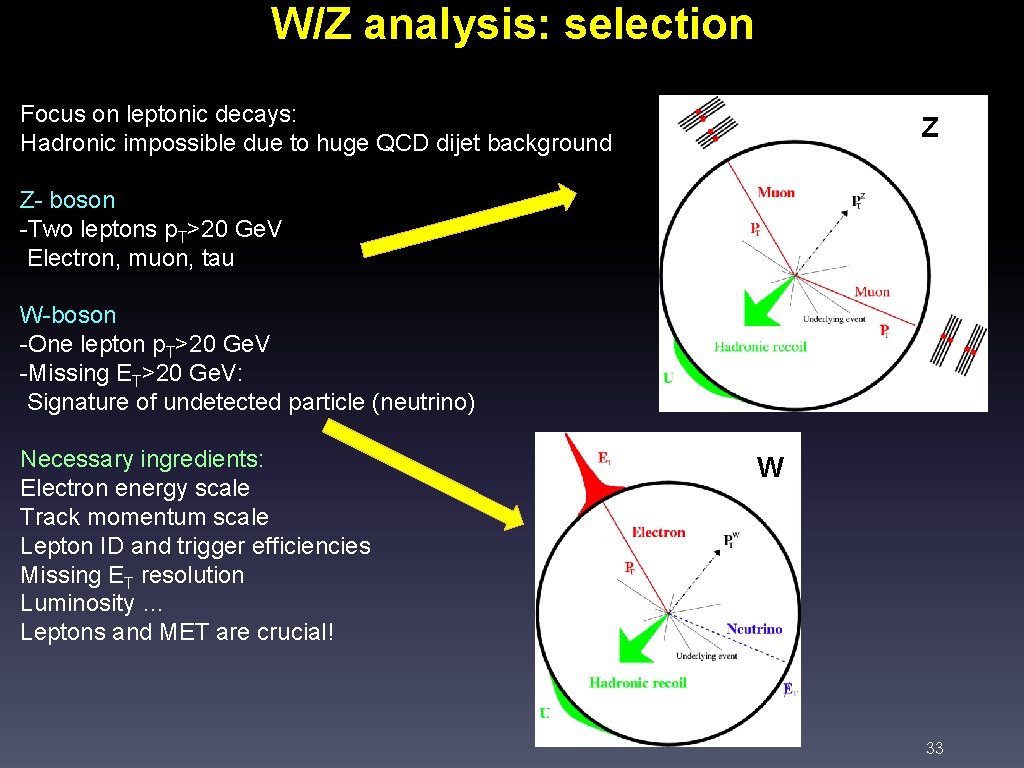
W/Z analysis: selection Focus on leptonic decays: Hadronic impossible due to huge QCD dijet background Z Z- boson -Two leptons p. T>20 Ge. V Electron, muon, tau W-boson -One lepton p. T>20 Ge. V -Missing ET>20 Ge. V: Signature of undetected particle (neutrino) Necessary ingredients: Electron energy scale Track momentum scale Lepton ID and trigger efficiencies Missing ET resolution Luminosity … Leptons and MET are crucial! W 33
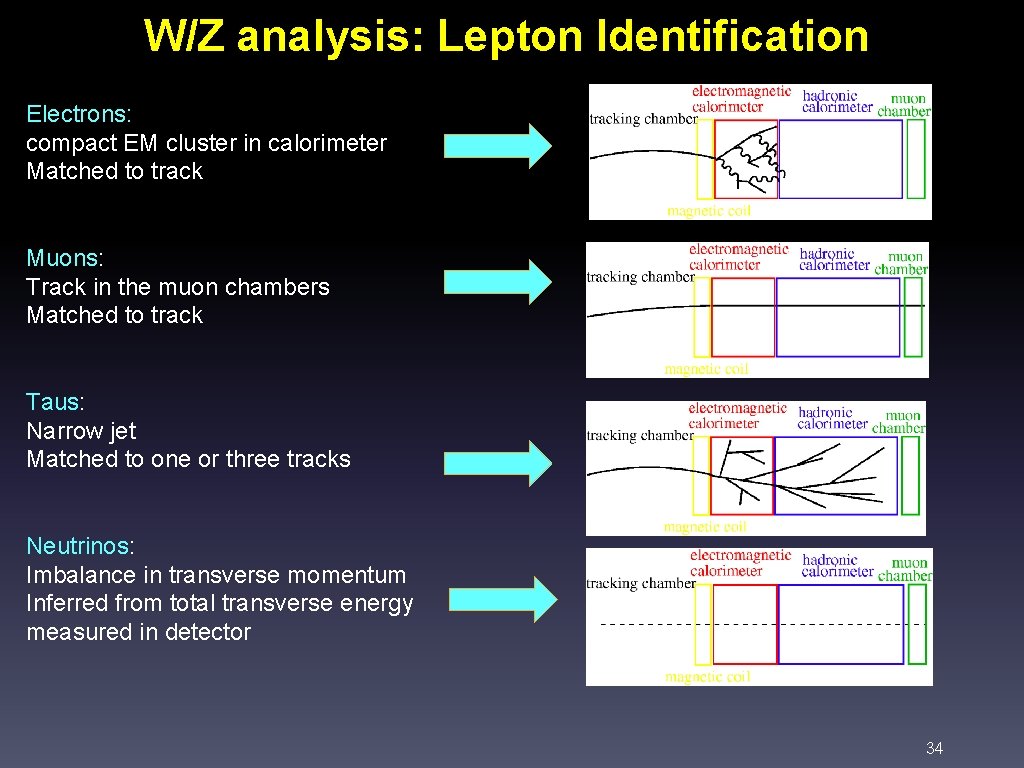
W/Z analysis: Lepton Identification Electrons: compact EM cluster in calorimeter Matched to track Muons: Track in the muon chambers Matched to track Taus: Narrow jet Matched to one or three tracks Neutrinos: Imbalance in transverse momentum Inferred from total transverse energy measured in detector 34
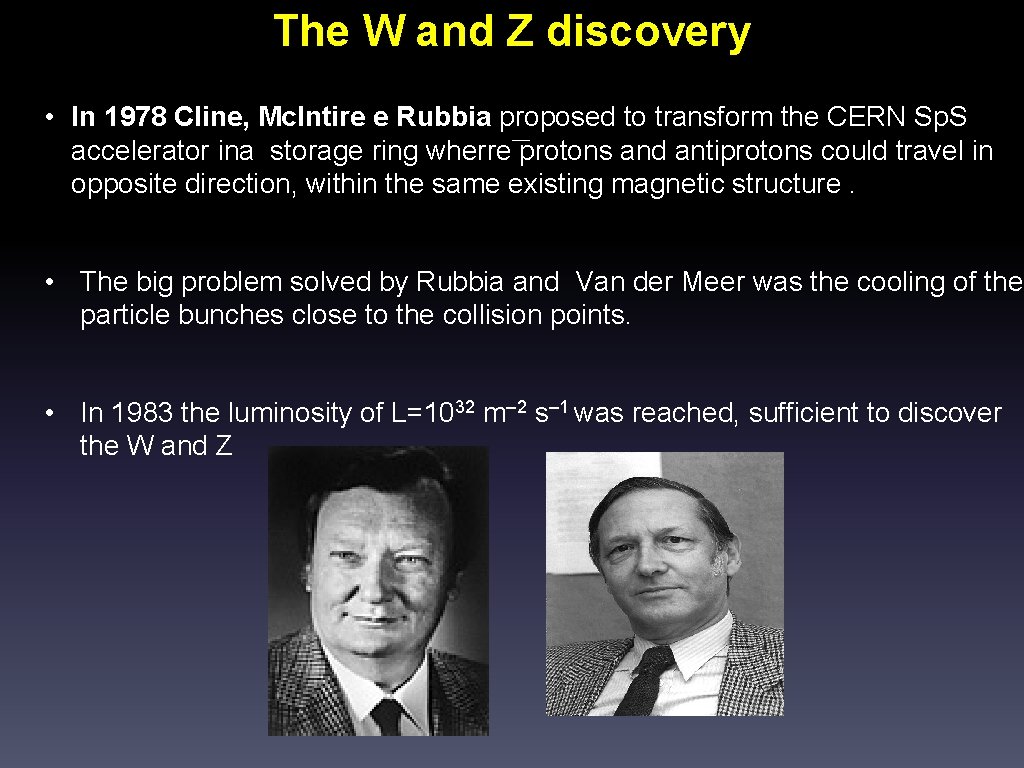
The W and Z discovery • In 1978 Cline, Mc. Intire e Rubbia proposed to transform the CERN Sp. S accelerator ina storage ring wherre protons and antiprotons could travel in opposite direction, within the same existing magnetic structure. • The big problem solved by Rubbia and Van der Meer was the cooling of the particle bunches close to the collision points. • In 1983 the luminosity of L=1032 m– 2 s– 1 was reached, sufficient to discover the W and Z
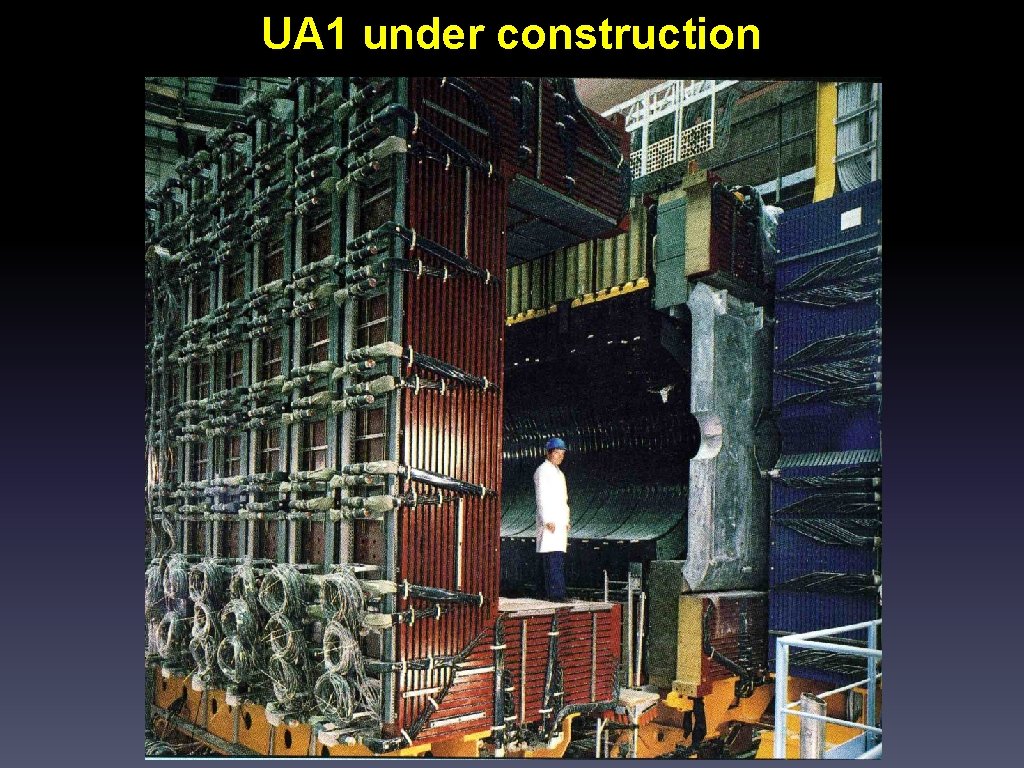
UA 1 under construction
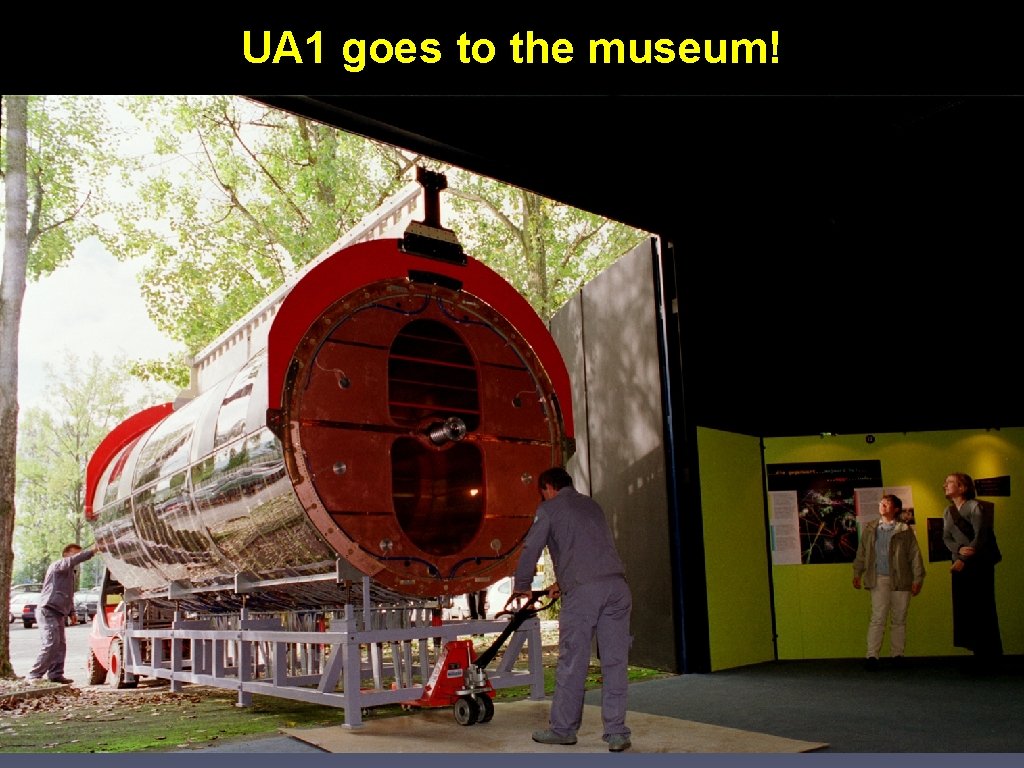
UA 1 goes to the museum! 6/5/2021
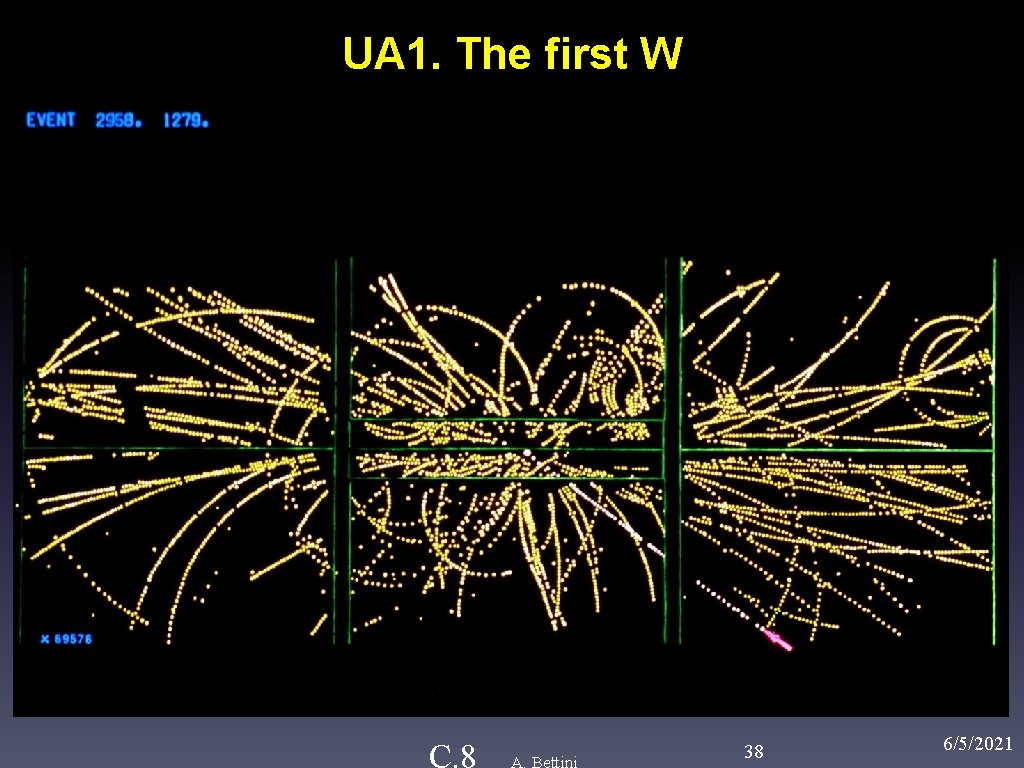
UA 1. The first W C. 8 A. Bettini 38 6/5/2021
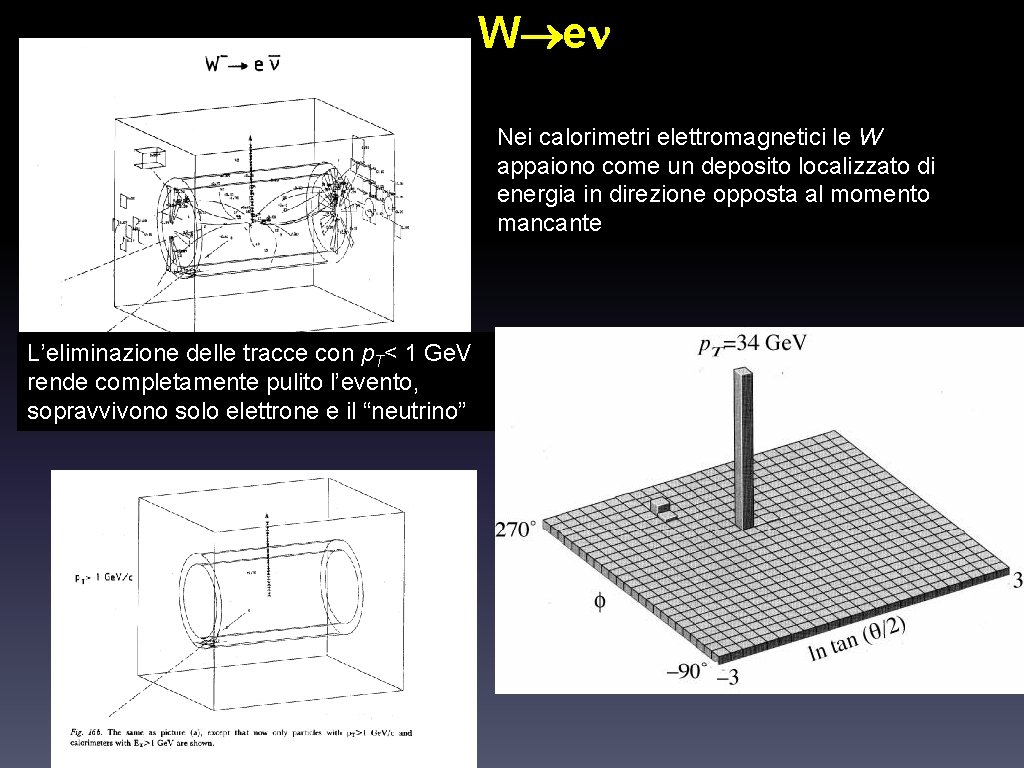
W e Nei calorimetri elettromagnetici le W appaiono come un deposito localizzato di energia in direzione opposta al momento mancante L’eliminazione delle tracce con p. T< 1 Ge. V rende completamente pulito l’evento, sopravvivono solo elettrone e il “neutrino”
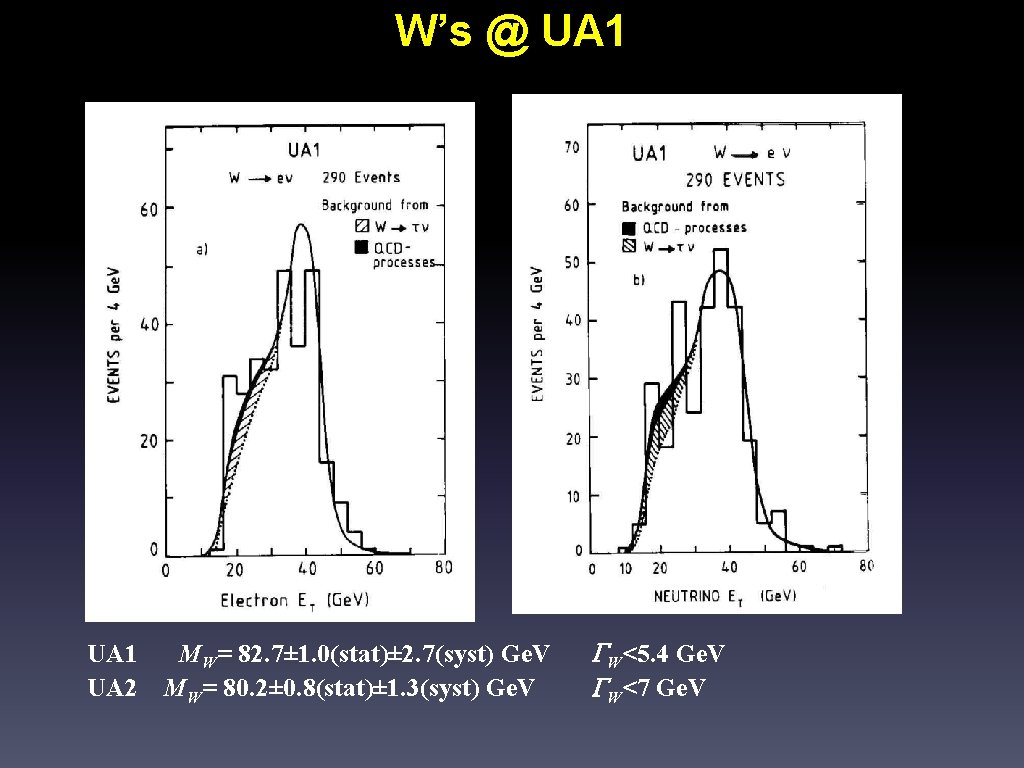
W’s @ UA 1 UA 2 MW= 82. 7± 1. 0(stat)± 2. 7(syst) Ge. V MW= 80. 2± 0. 8(stat)± 1. 3(syst) Ge. V GW<5. 4 Ge. V GW<7 Ge. V
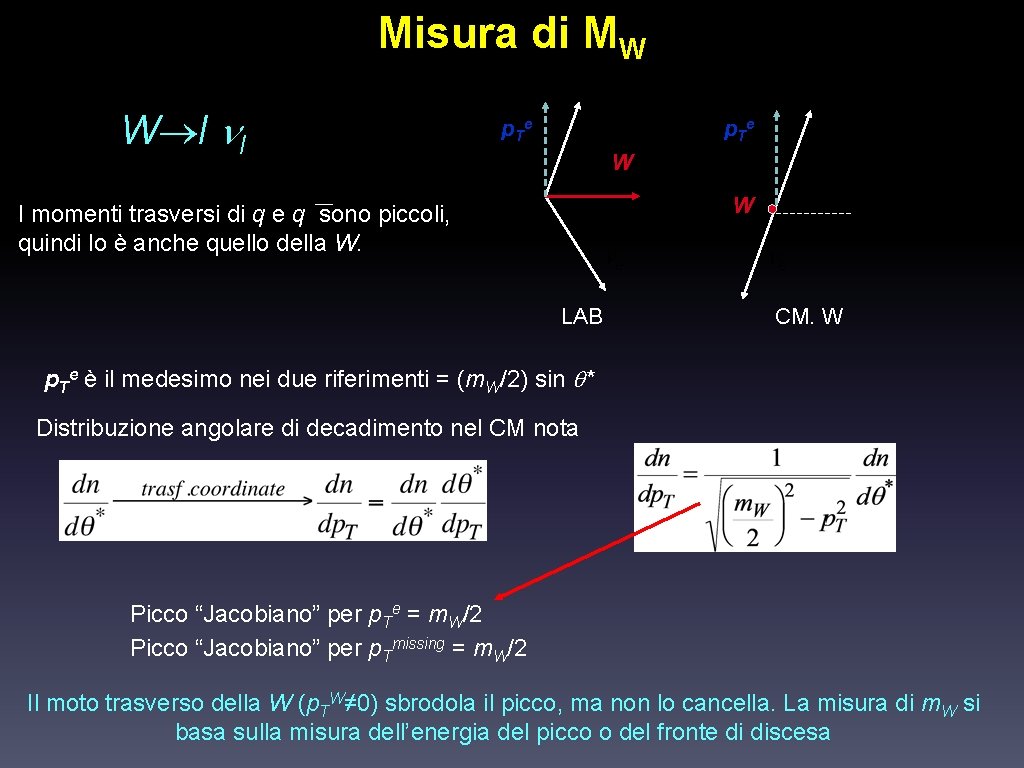
Misura di MW W l nl p. T e e e p Te W q* W I momenti trasversi di q e q sono piccoli, quindi lo è anche quello della W. ne LAB pe = m. W/2 ne CM. W p. Te è il medesimo nei due riferimenti = (m. W/2) sin q* Distribuzione angolare di decadimento nel CM nota Picco “Jacobiano” per p. Te = m. W/2 Picco “Jacobiano” per p. Tmissing = m. W/2 Il moto trasverso della W (p. TW≠ 0) sbrodola il picco, ma non lo cancella. La misura di m. W si basa sulla misura dell’energia del picco o del fronte di discesa
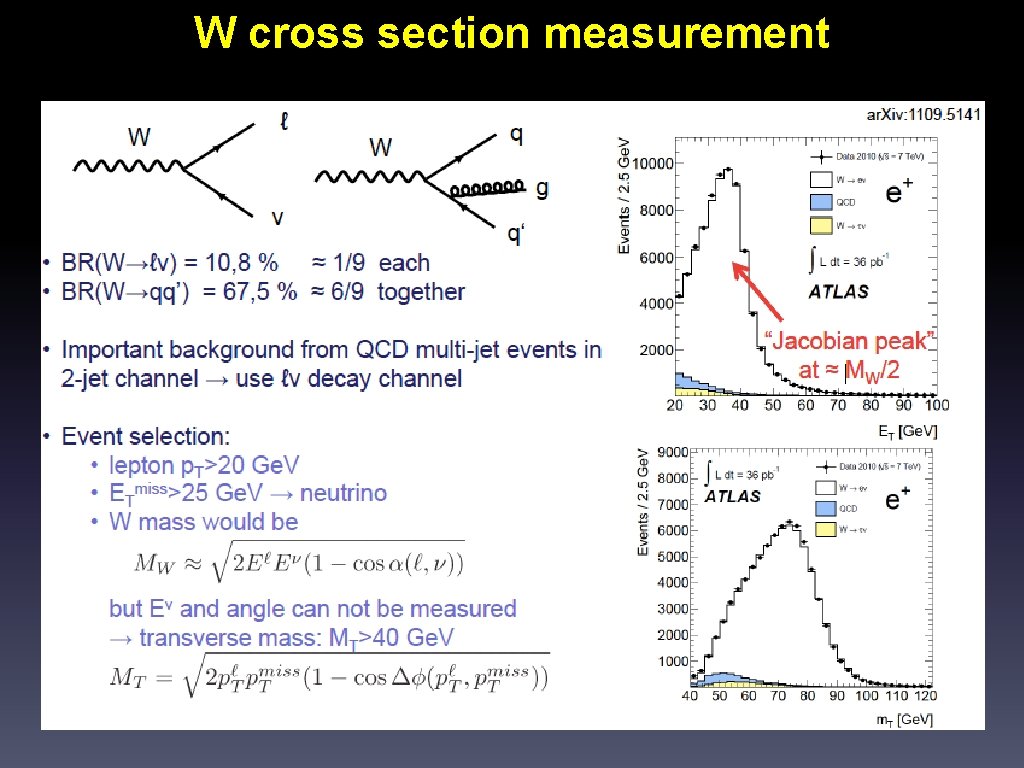
W cross section measurement
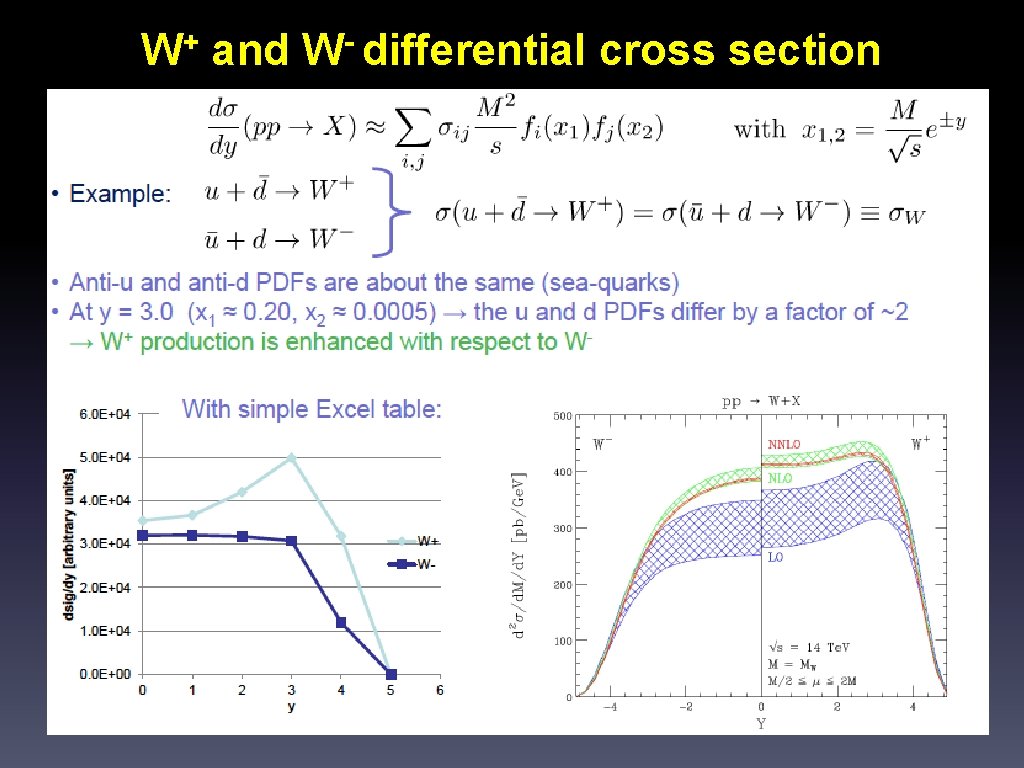
+ W and W differential cross section
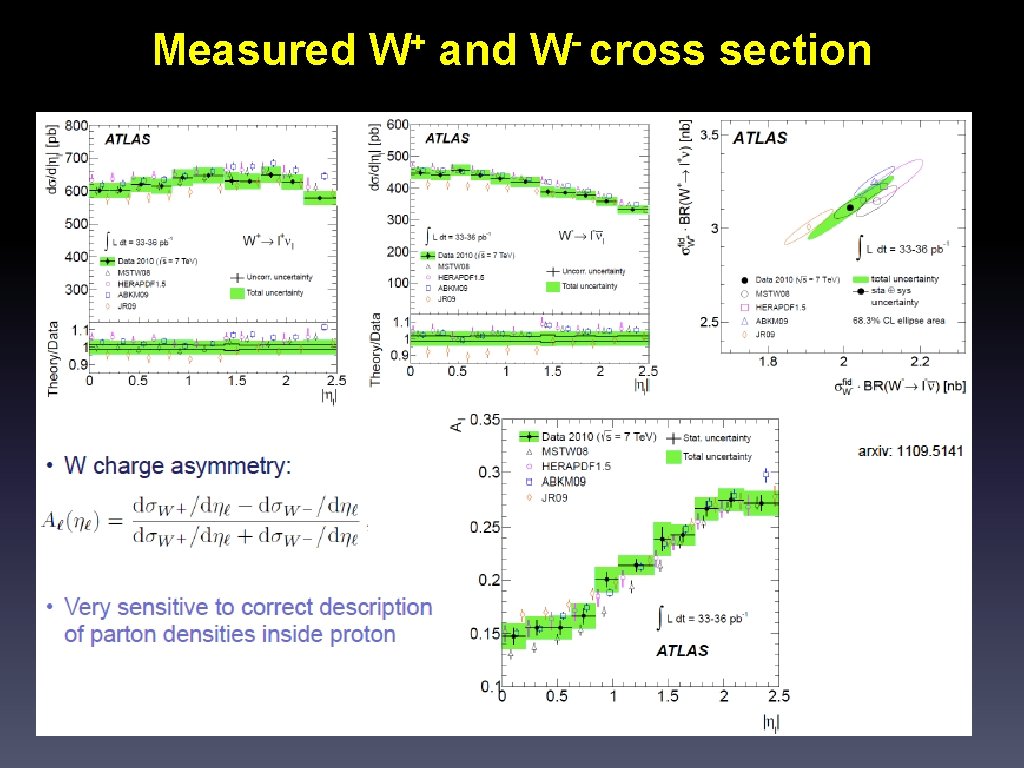
Measured + W and W cross section
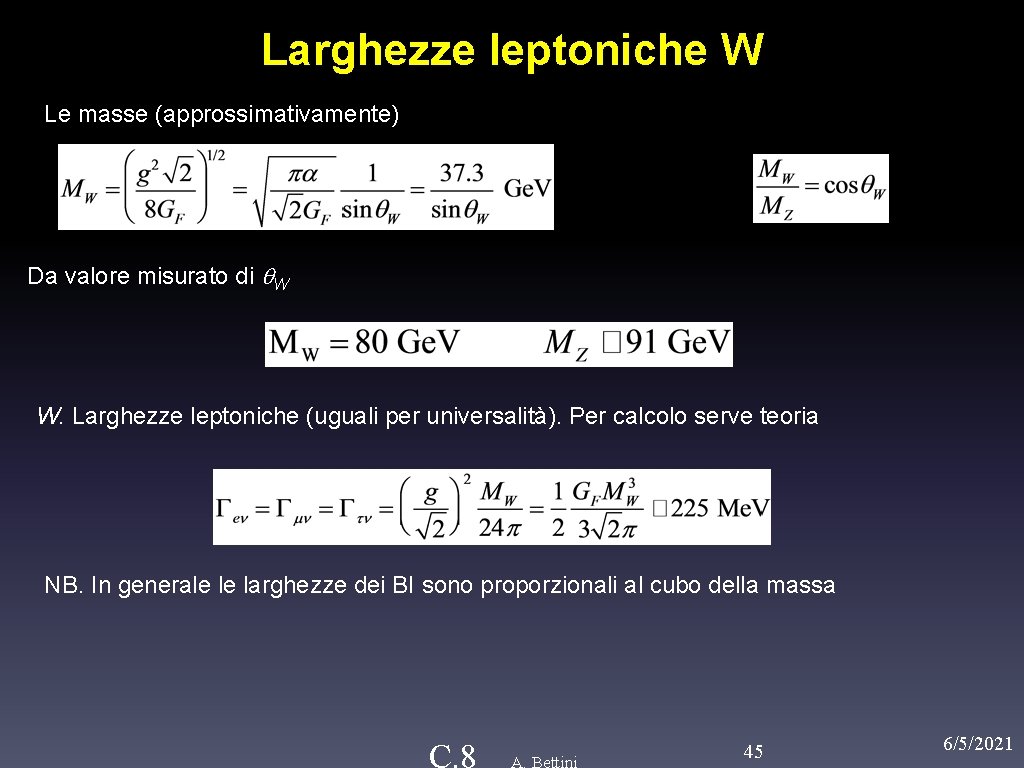
Larghezze leptoniche W Le masse (approssimativamente) Da valore misurato di q. W W. Larghezze leptoniche (uguali per universalità). Per calcolo serve teoria NB. In generale le larghezze dei BI sono proporzionali al cubo della massa C. 8 A. Bettini 45 6/5/2021
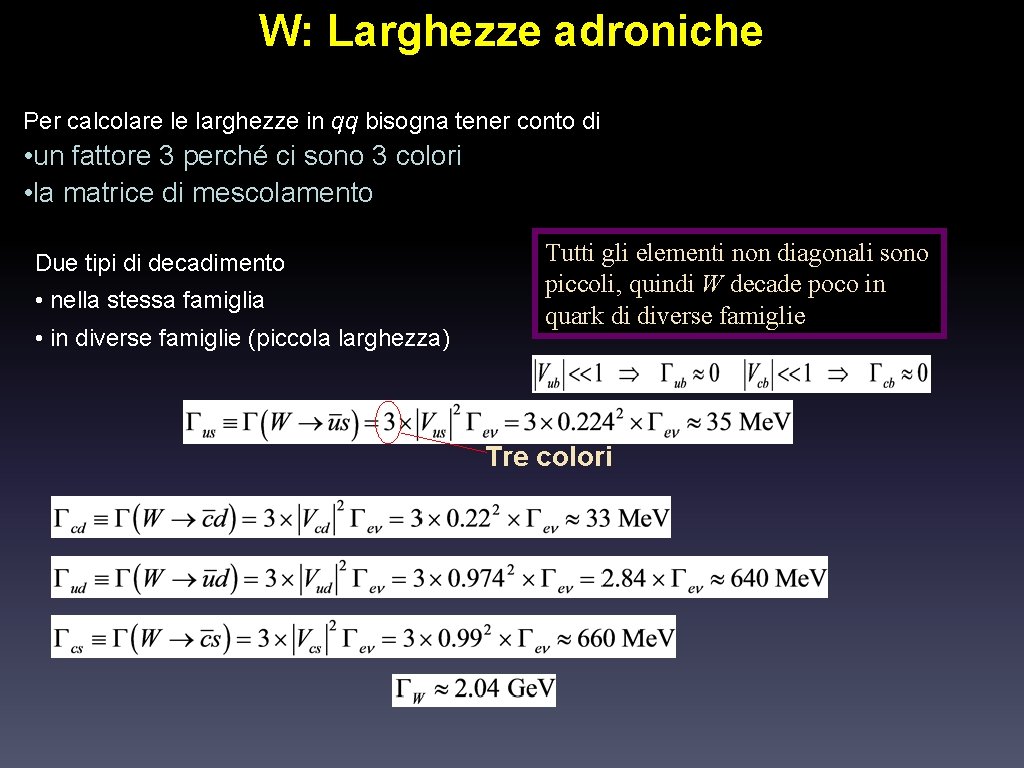
W: Larghezze adroniche Per calcolare le larghezze in qq bisogna tener conto di • un fattore 3 perché ci sono 3 colori • la matrice di mescolamento Due tipi di decadimento • nella stessa famiglia • in diverse famiglie (piccola larghezza) Tutti gli elementi non diagonali sono piccoli, quindi W decade poco in quark di diverse famiglie Tre colori
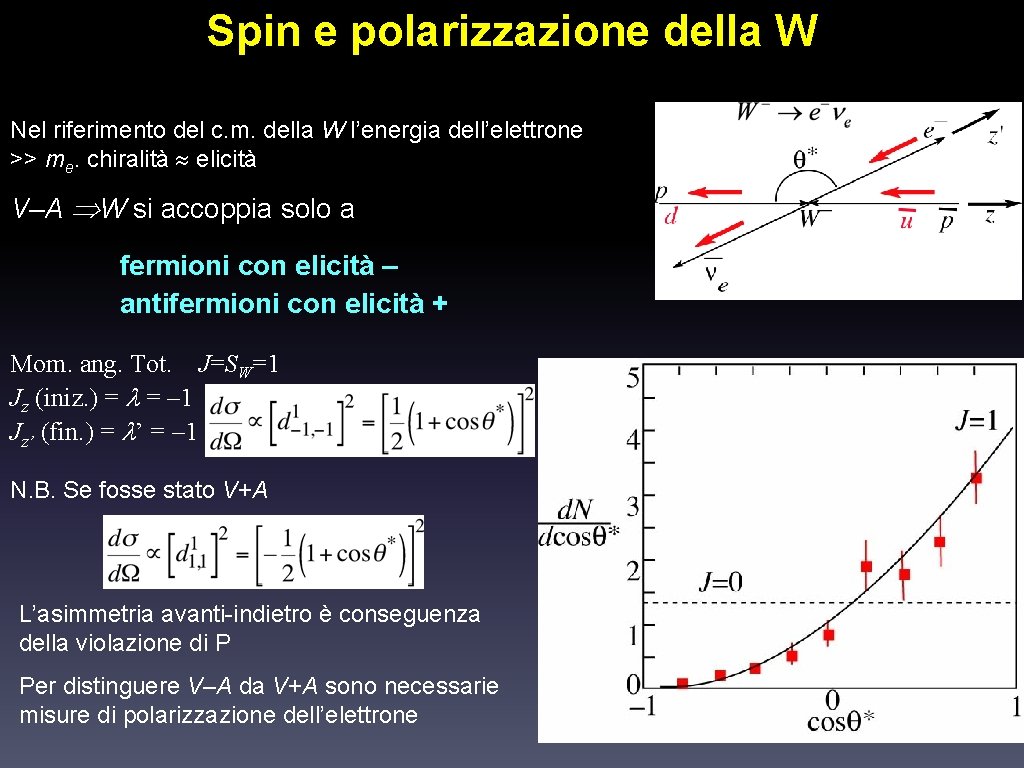
Spin e polarizzazione della W Nel riferimento del c. m. della W l’energia dell’elettrone >> me. chiralità elicità V–A W si accoppia solo a fermioni con elicità – antifermioni con elicità + Mom. ang. Tot. J=SW=1 Jz (iniz. ) = l = – 1 Jz’ (fin. ) = l’ = – 1 N. B. Se fosse stato V+A L’asimmetria avanti-indietro è conseguenza della violazione di P Per distinguere V–A da V+A sono necessarie misure di polarizzazione dell’elettrone
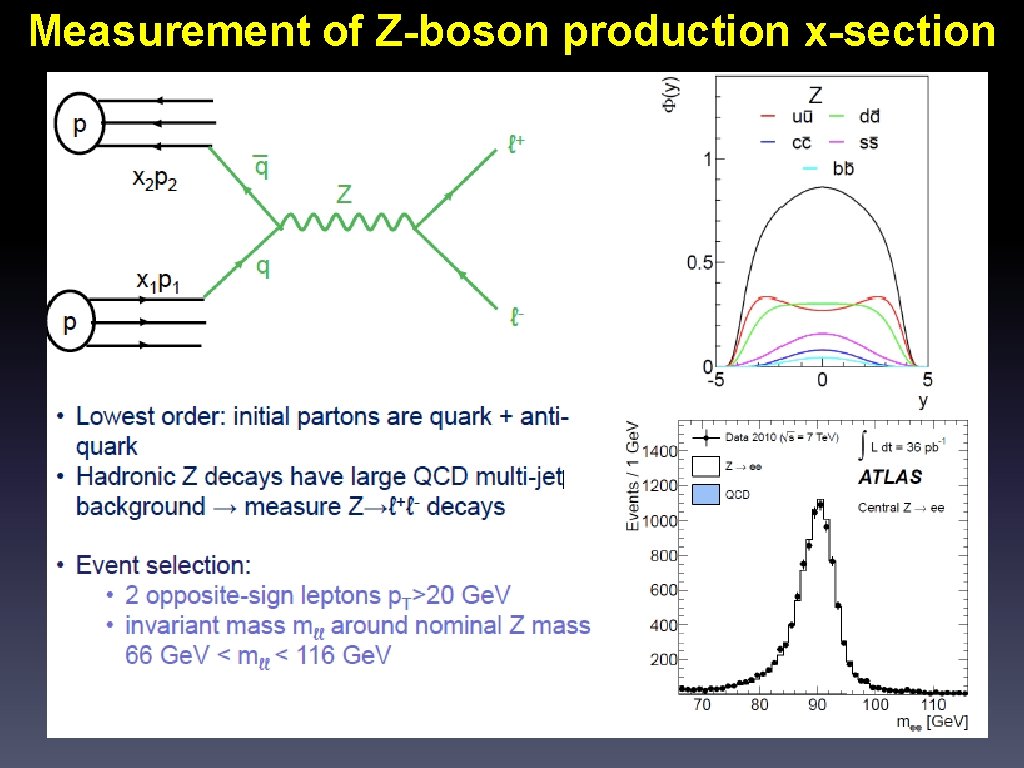
Measurement of Z-boson production x-section
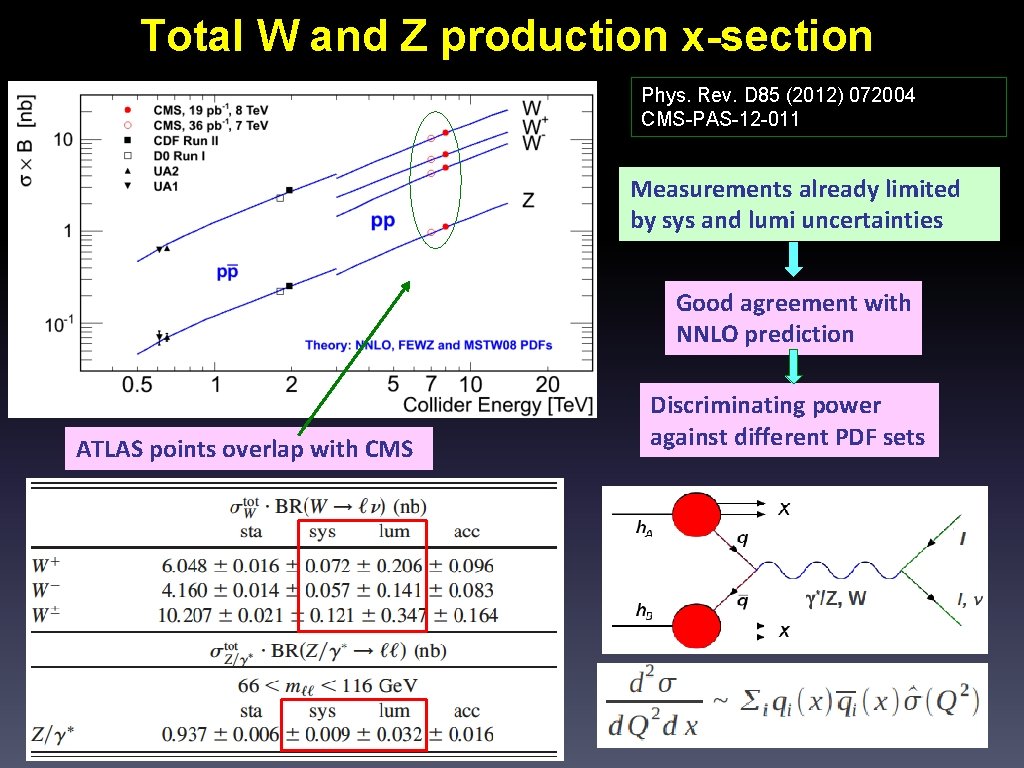
Total W and Z production x-section Phys. Rev. D 85 (2012) 072004 CMS-PAS-12 -011 Measurements already limited by sys and lumi uncertainties Good agreement with NNLO prediction ATLAS points overlap with CMS X. Wu, SUSY 2012, 13/08/12 Discriminating power against different PDF sets 49
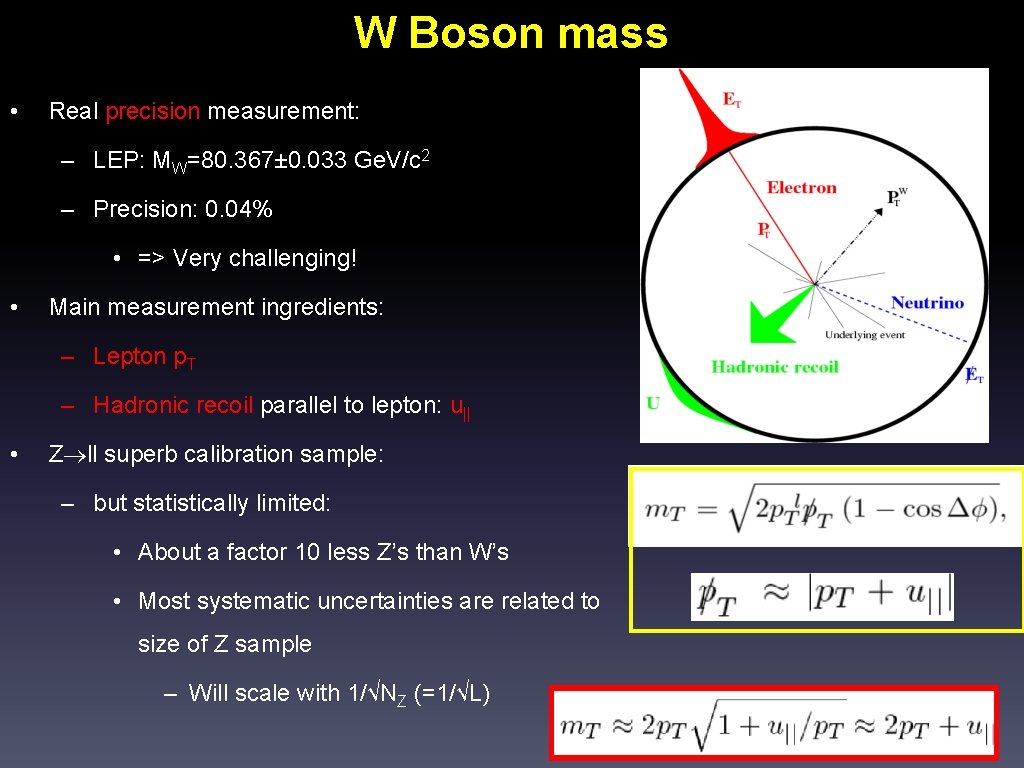
W Boson mass • Real precision measurement: – LEP: MW=80. 367± 0. 033 Ge. V/c 2 – Precision: 0. 04% • => Very challenging! • Main measurement ingredients: – Lepton p. T – Hadronic recoil parallel to lepton: u|| • Z ll superb calibration sample: – but statistically limited: • About a factor 10 less Z’s than W’s • Most systematic uncertainties are related to size of Z sample – Will scale with 1/√NZ (=1/√L) 50
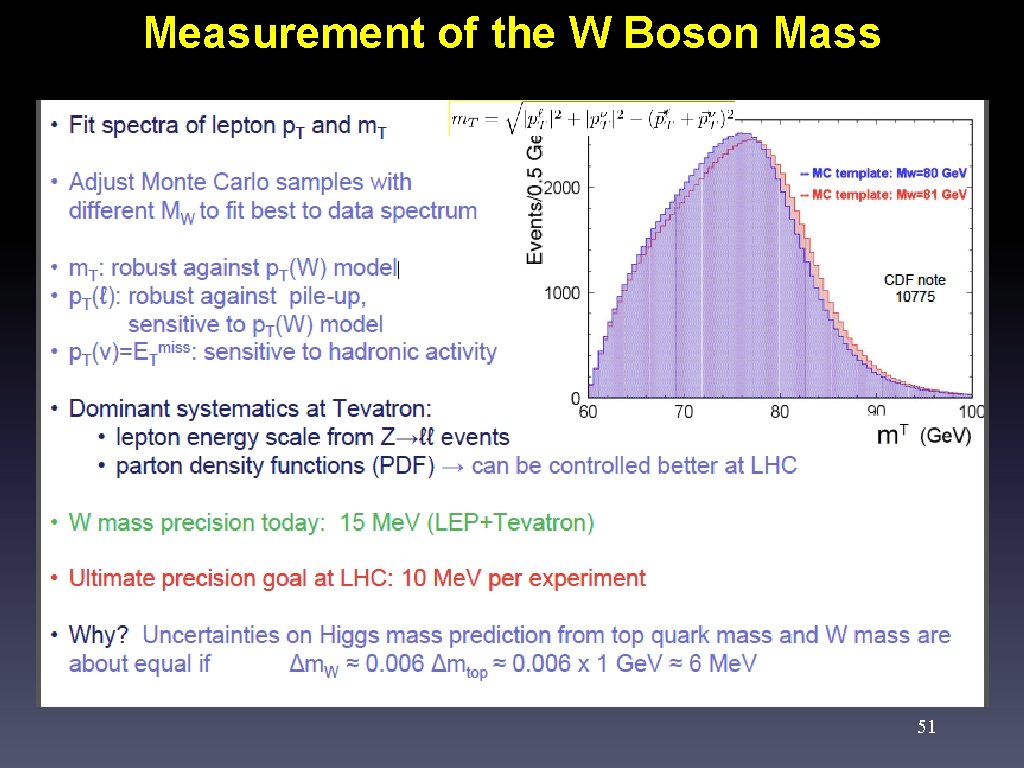
Measurement of the W Boson Mass 51
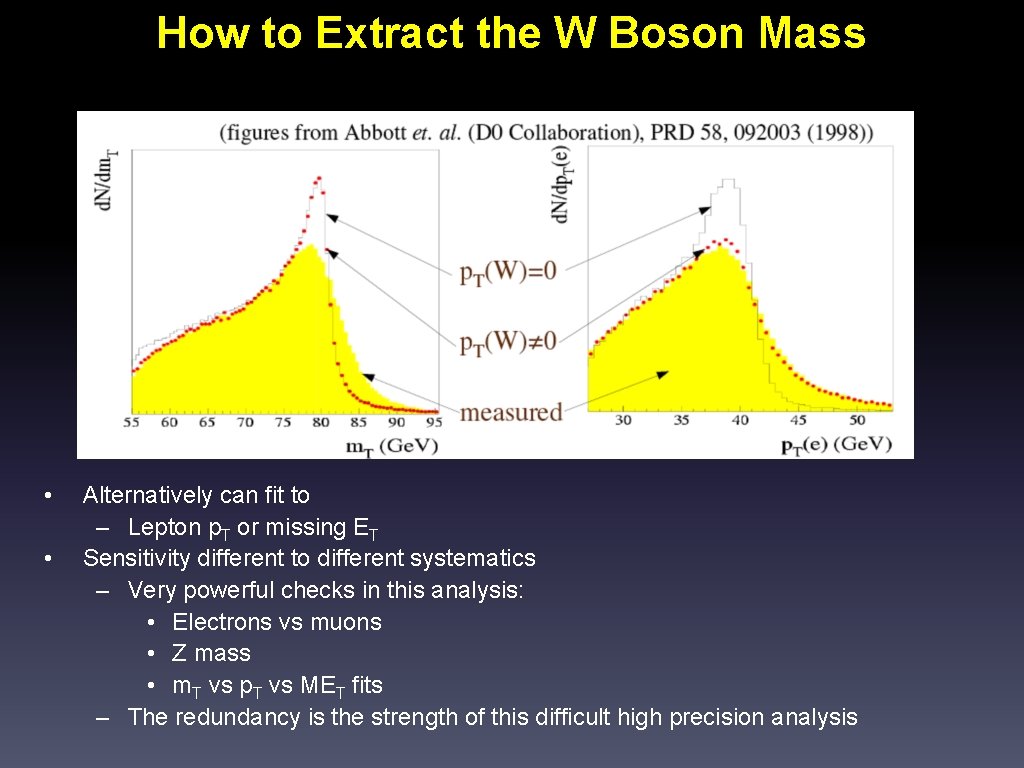
How to Extract the W Boson Mass • • Alternatively can fit to – Lepton p. T or missing ET Sensitivity different to different systematics – Very powerful checks in this analysis: • Electrons vs muons • Z mass • m. T vs p. T vs MET fits – The redundancy is the strength of this difficult high precision analysis
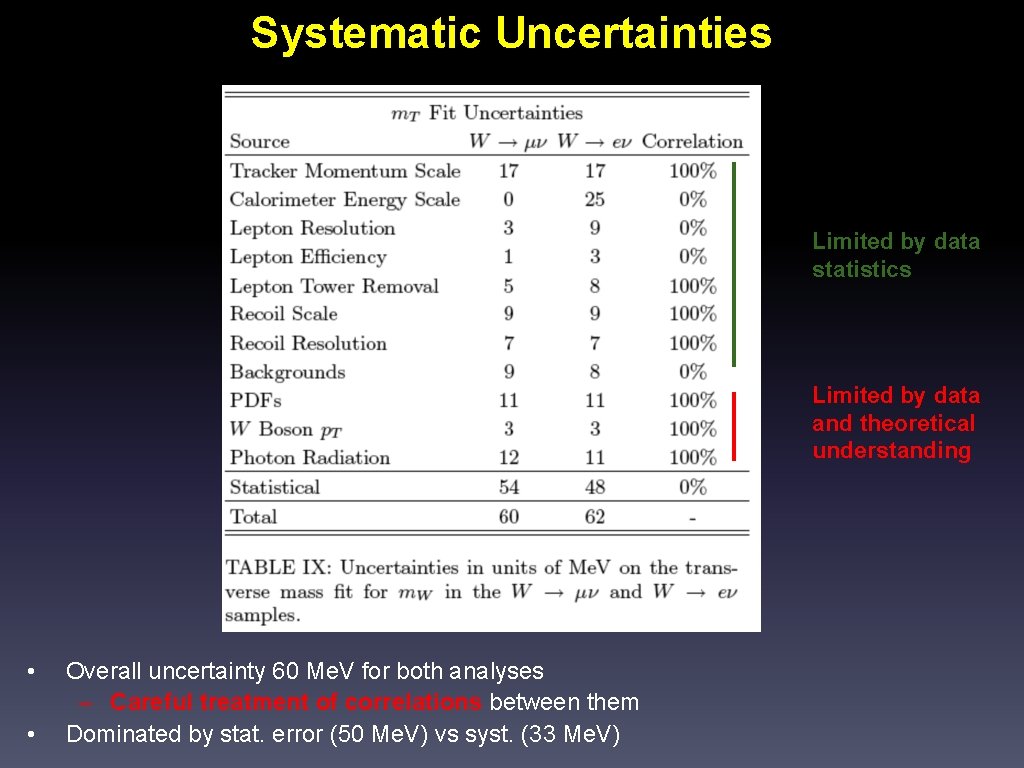
Systematic Uncertainties Limited by data statistics Limited by data and theoretical understanding • • Overall uncertainty 60 Me. V for both analyses – Careful treatment of correlations between them Dominated by stat. error (50 Me. V) vs syst. (33 Me. V)
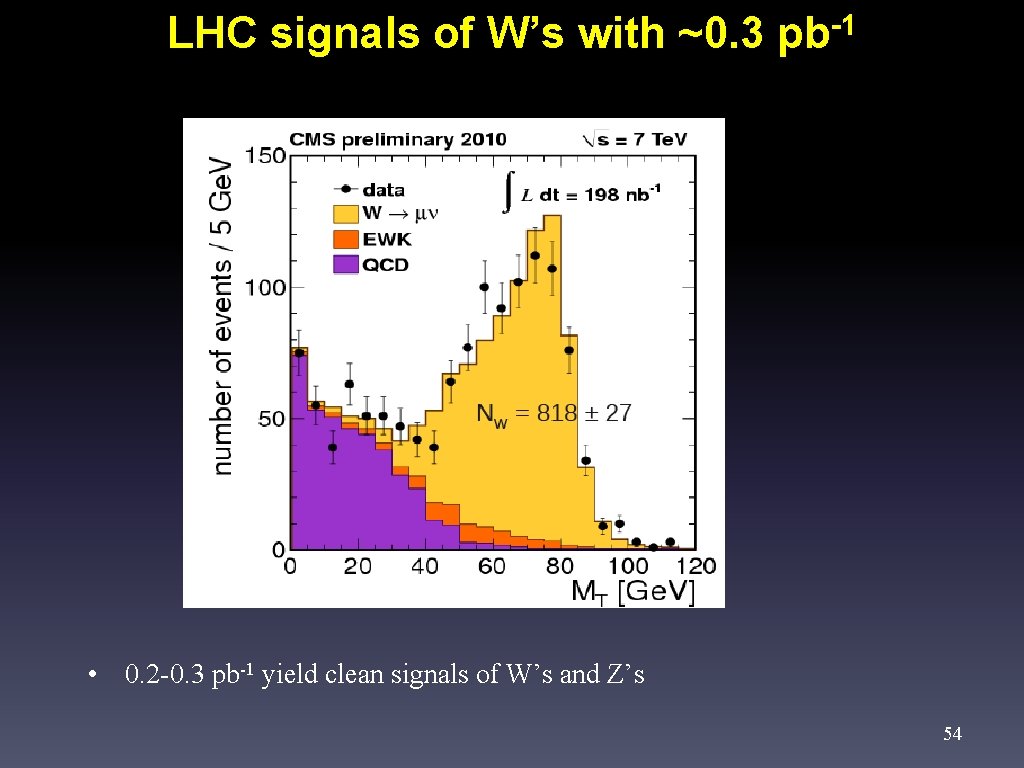
LHC signals of W’s with ~0. 3 pb-1 • 0. 2 -0. 3 pb-1 yield clean signals of W’s and Z’s 54
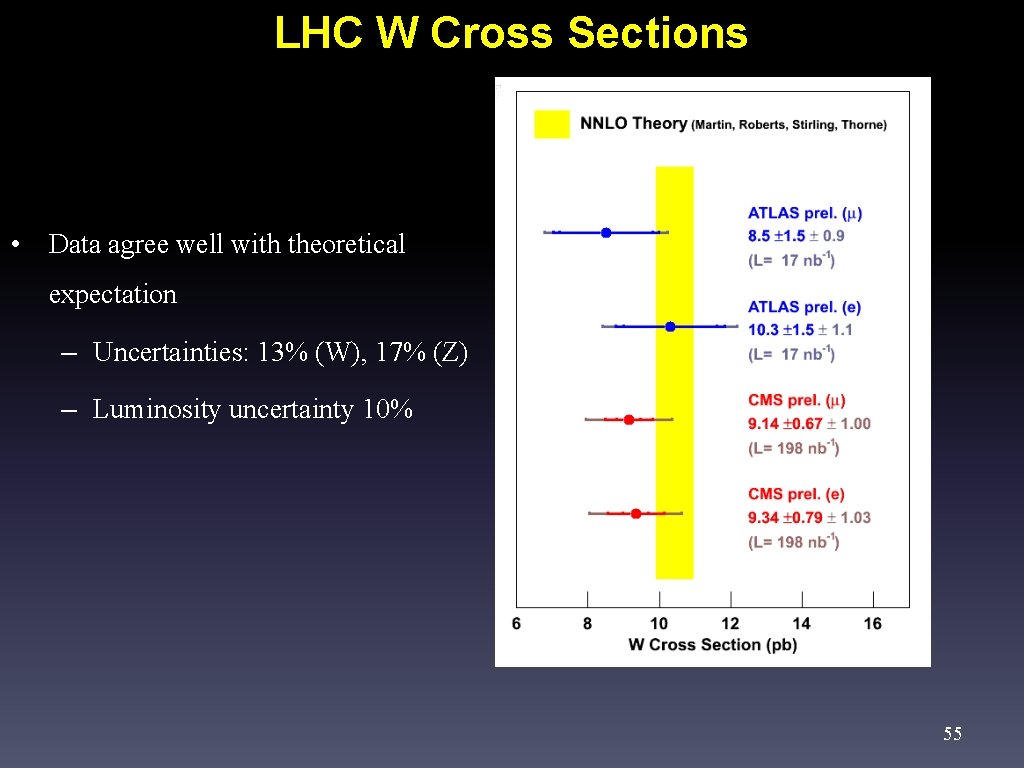
LHC W Cross Sections • Data agree well with theoretical expectation – Uncertainties: 13% (W), 17% (Z) – Luminosity uncertainty 10% 55
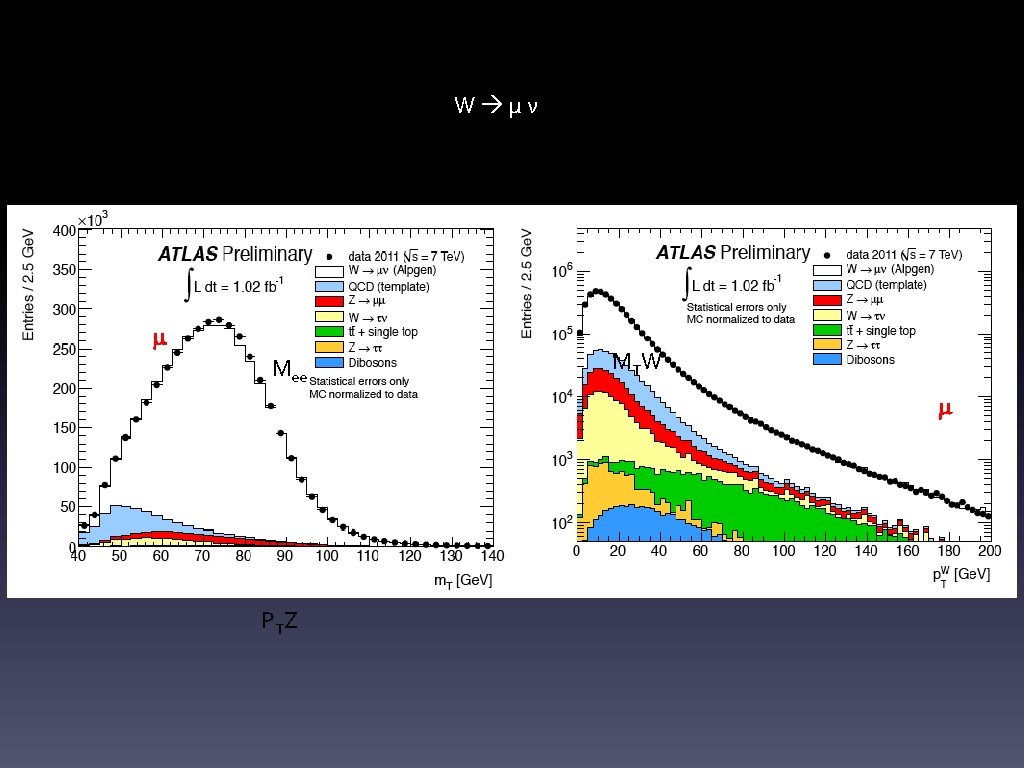
W μν m Mee M TW m P TZ
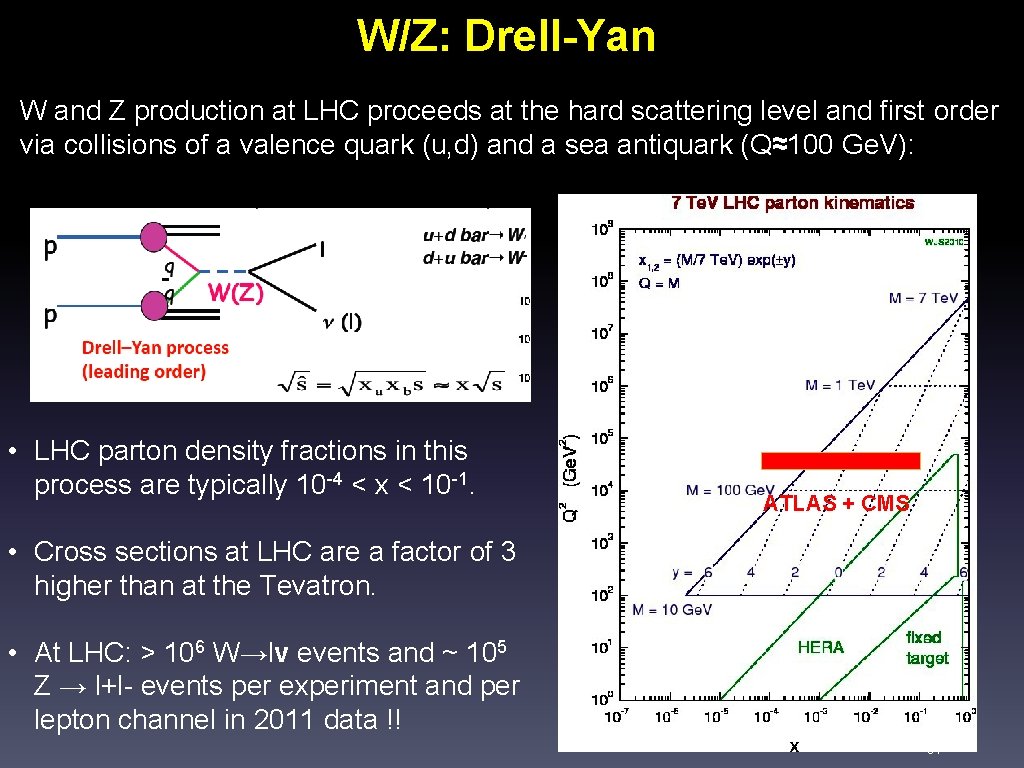
W/Z: Drell-Yan W and Z production at LHC proceeds at the hard scattering level and first order via collisions of a valence quark (u, d) and a sea antiquark (Q≈100 Ge. V): • LHC parton density fractions in this process are typically 10 -4 < x < 10 -1. ATLAS + CMS • Cross sections at LHC are a factor of 3 higher than at the Tevatron. • At LHC: > 106 W→lν events and ~ 105 Z → l+l- events per experiment and per lepton channel in 2011 data !! 57
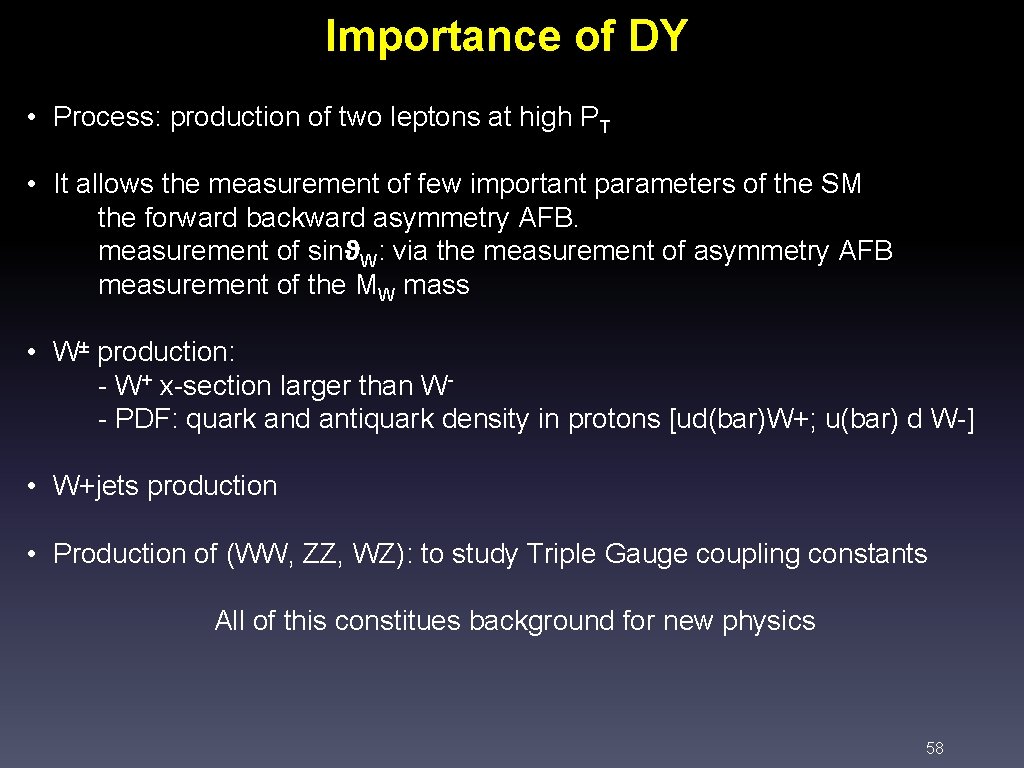
Importance of DY • Process: production of two leptons at high PT • It allows the measurement of few important parameters of the SM the forward backward asymmetry AFB. measurement of sinϑW: via the measurement of asymmetry AFB measurement of the MW mass • W± production: - W+ x-section larger than W- PDF: quark and antiquark density in protons [ud(bar)W+; u(bar) d W-] • W+jets production • Production of (WW, ZZ, WZ): to study Triple Gauge coupling constants All of this constitues background for new physics 58
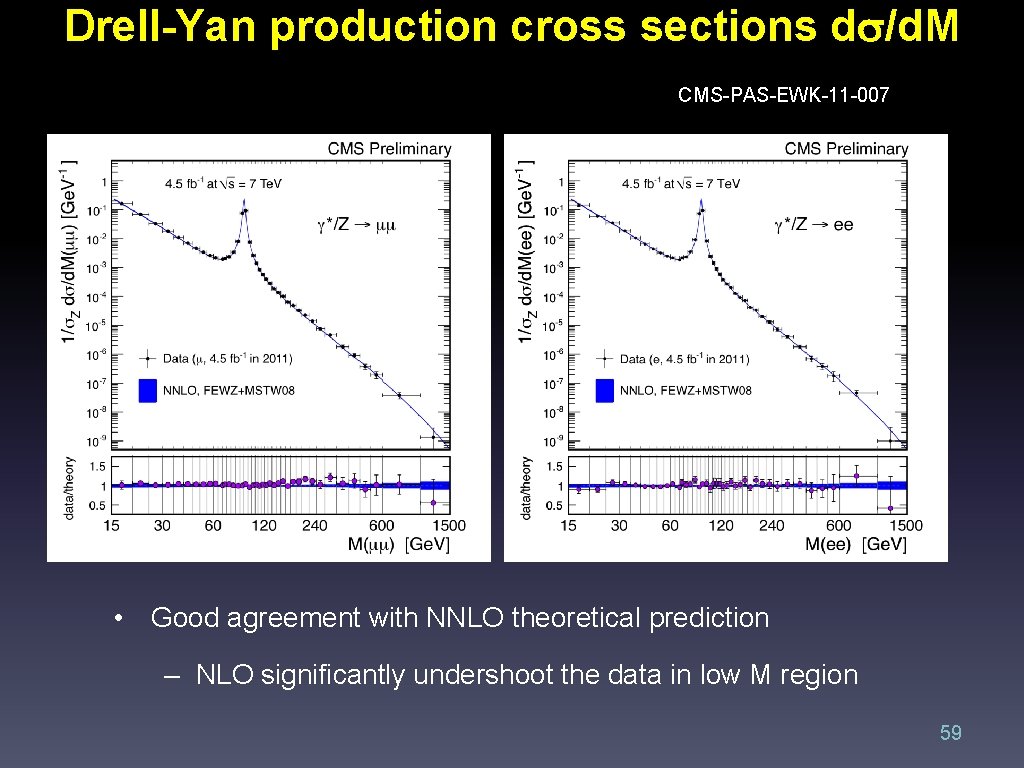
Drell-Yan production cross sections ds/d. M CMS-PAS-EWK-11 -007 • Good agreement with NNLO theoretical prediction – NLO significantly undershoot the data in low M region 59
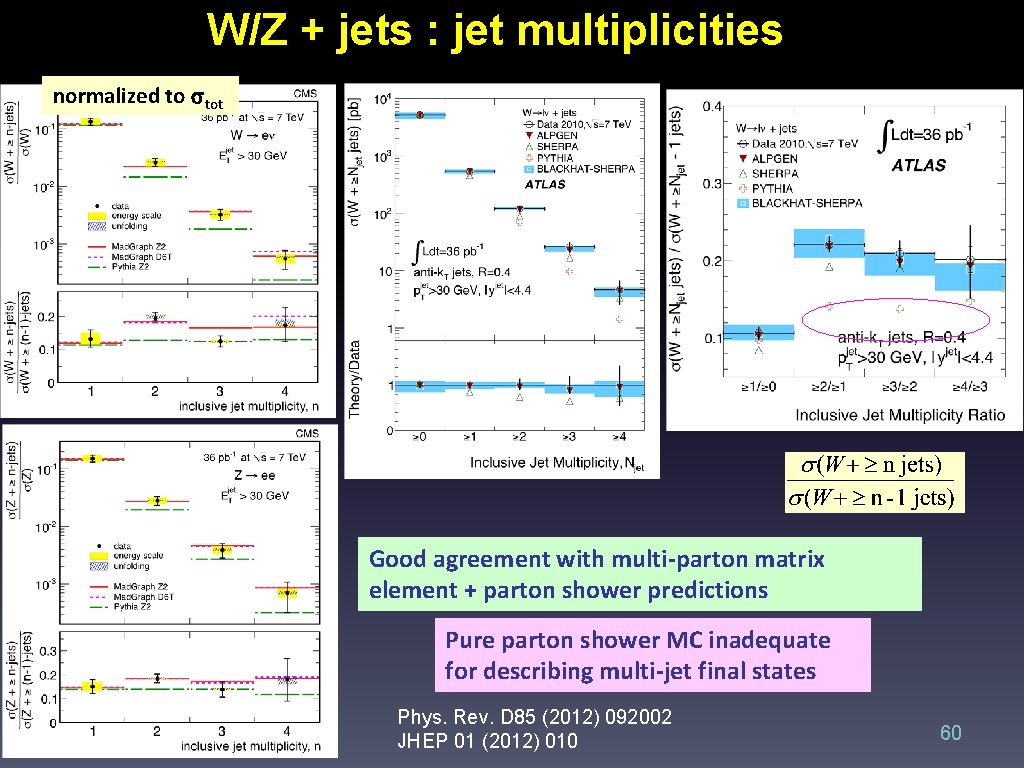
W/Z + jets : jet multiplicities normalized to stot Good agreement with multi-parton matrix element + parton shower predictions Pure parton shower MC inadequate for describing multi-jet final states X. Wu, SUSY 2012, 13/08/12 Phys. Rev. D 85 (2012) 092002 JHEP 01 (2012) 010 60
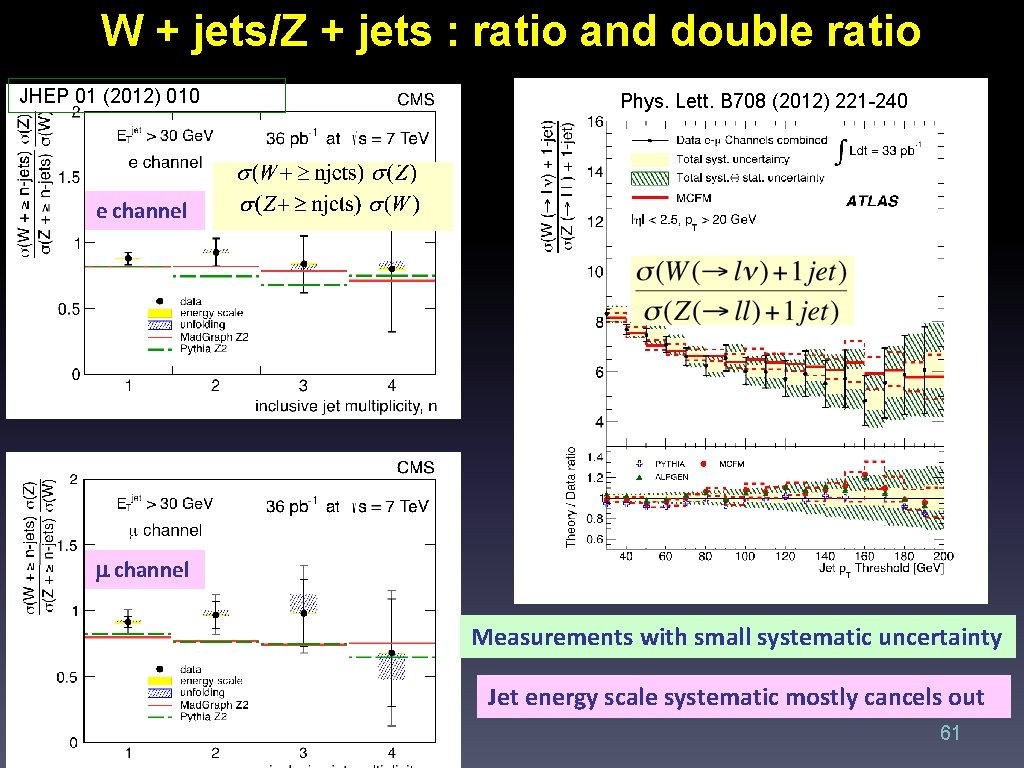
W + jets/Z + jets : ratio and double ratio JHEP 01 (2012) 010 Phys. Lett. B 708 (2012) 221 -240 e channel m channel Measurements with small systematic uncertainty Jet energy scale systematic mostly cancels out X. Wu, SUSY 2012, 13/08/12 61
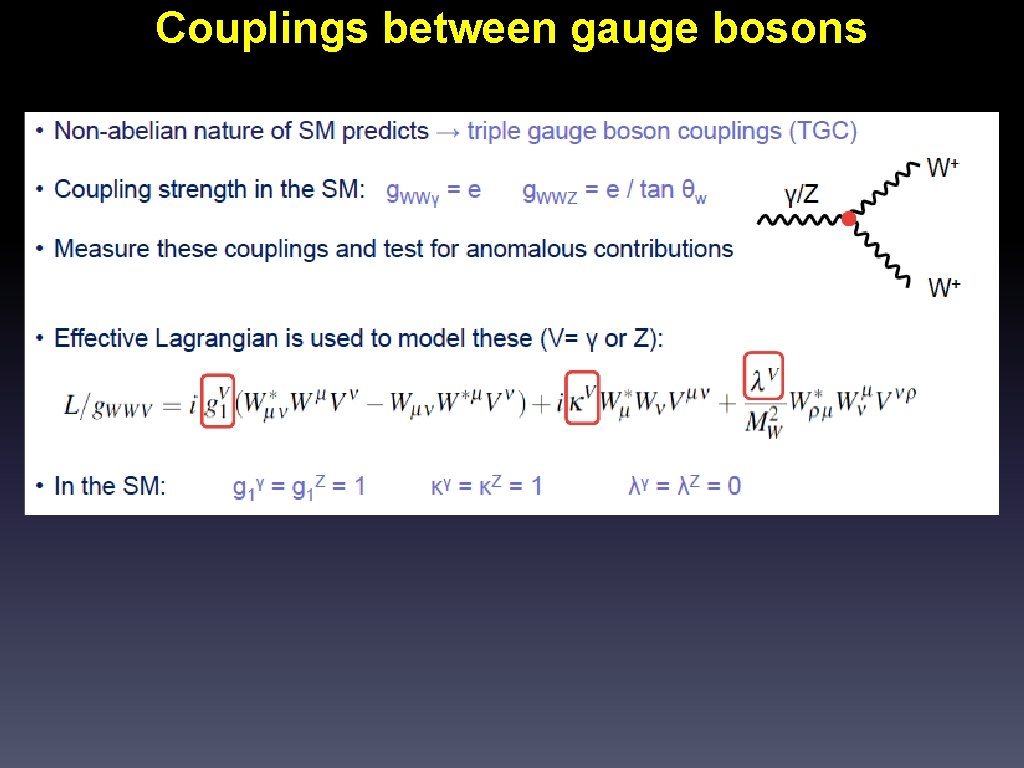
Couplings between gauge bosons
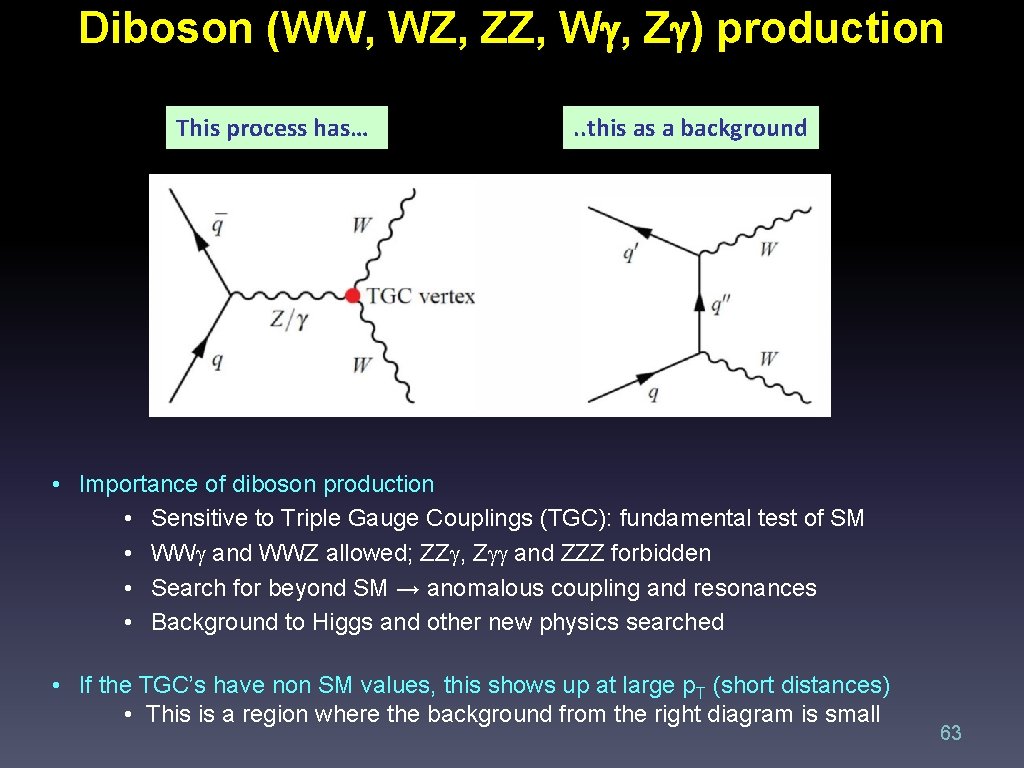
Diboson (WW, WZ, ZZ, W , Z ) production This process has… . . this as a background • Importance of diboson production • Sensitive to Triple Gauge Couplings (TGC): fundamental test of SM • WW and WWZ allowed; ZZ , Z and ZZZ forbidden • Search for beyond SM → anomalous coupling and resonances • Background to Higgs and other new physics searched • If the TGC’s have non SM values, this shows up at large p. T (short distances) • This is a region where the background from the right diagram is small 63
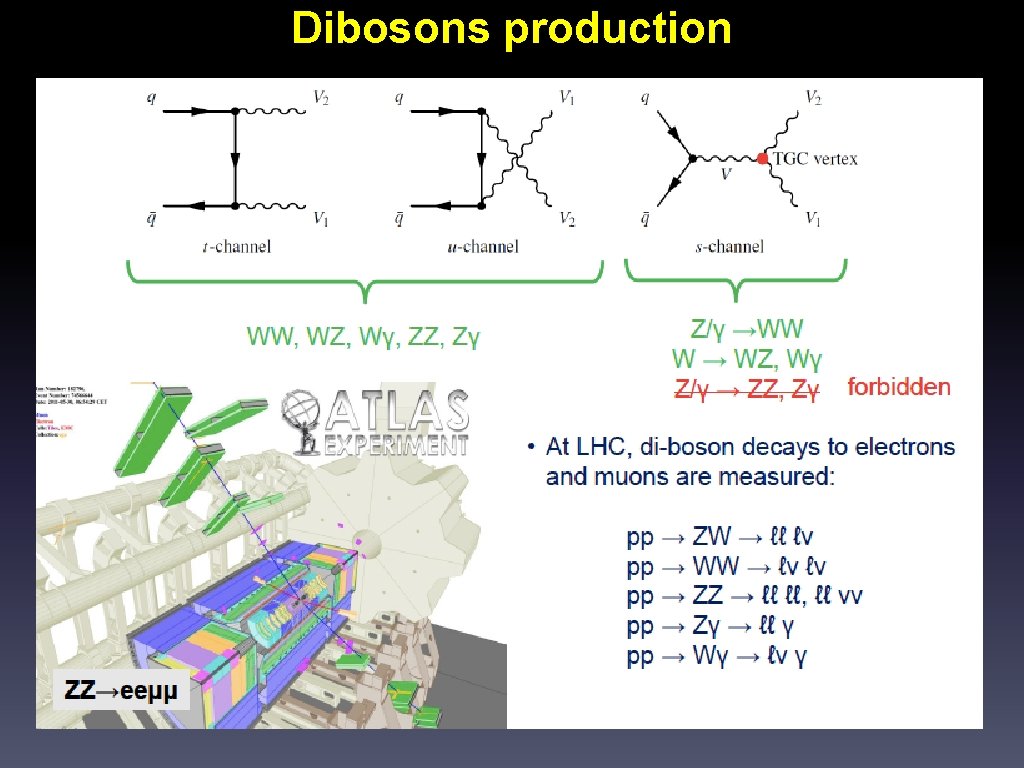
Dibosons production
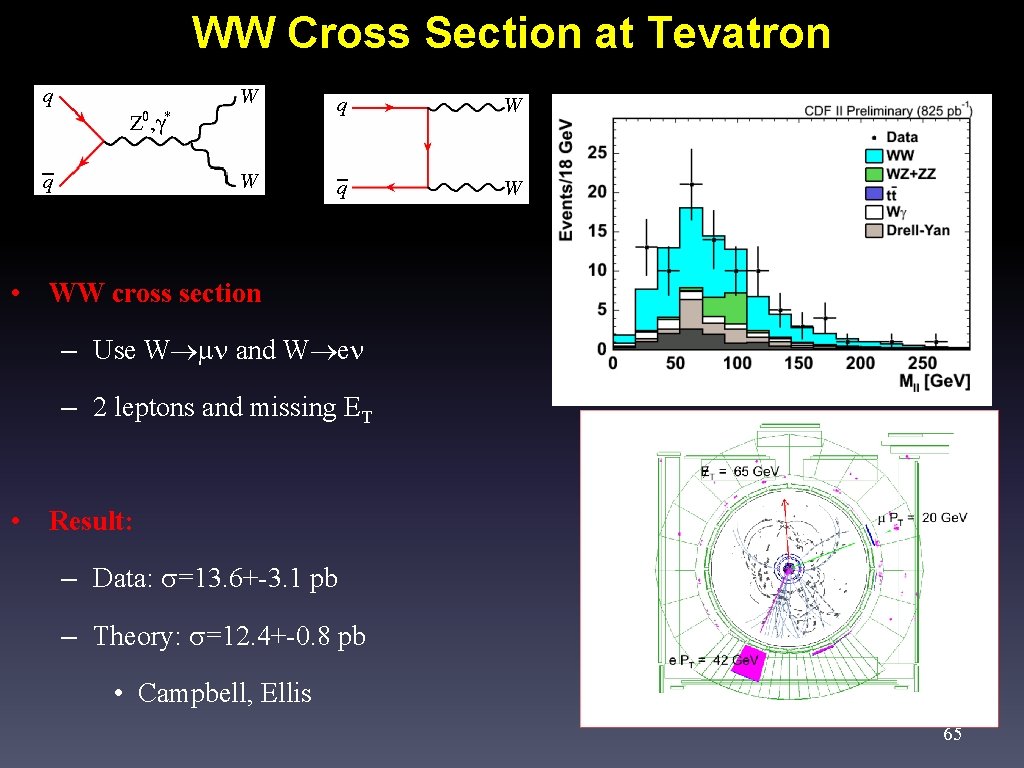
WW Cross Section at Tevatron • WW cross section – Use W and W e – 2 leptons and missing ET • Result: – Data: =13. 6+-3. 1 pb – Theory: =12. 4+-0. 8 pb • Campbell, Ellis 65
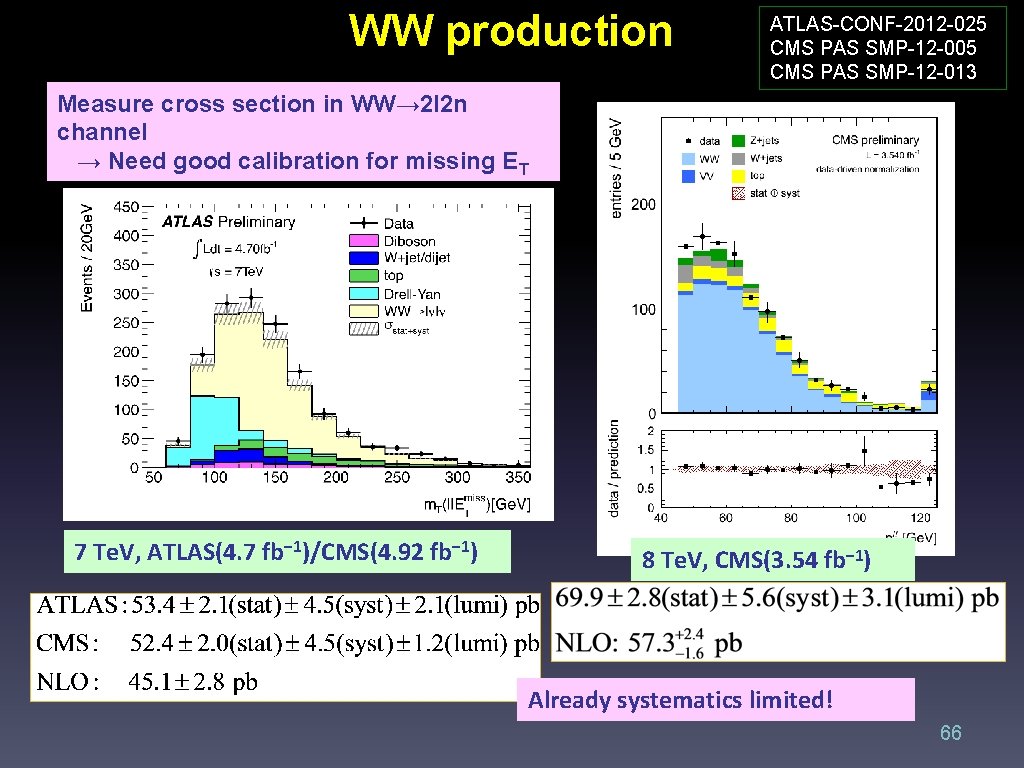
WW production ATLAS-CONF-2012 -025 CMS PAS SMP-12 -005 CMS PAS SMP-12 -013 Measure cross section in WW→ 2 l 2 n channel → Need good calibration for missing ET 7 Te. V, ATLAS(4. 7 fb− 1)/CMS(4. 92 fb− 1) 8 Te. V, CMS(3. 54 fb− 1) Already systematics limited! 66
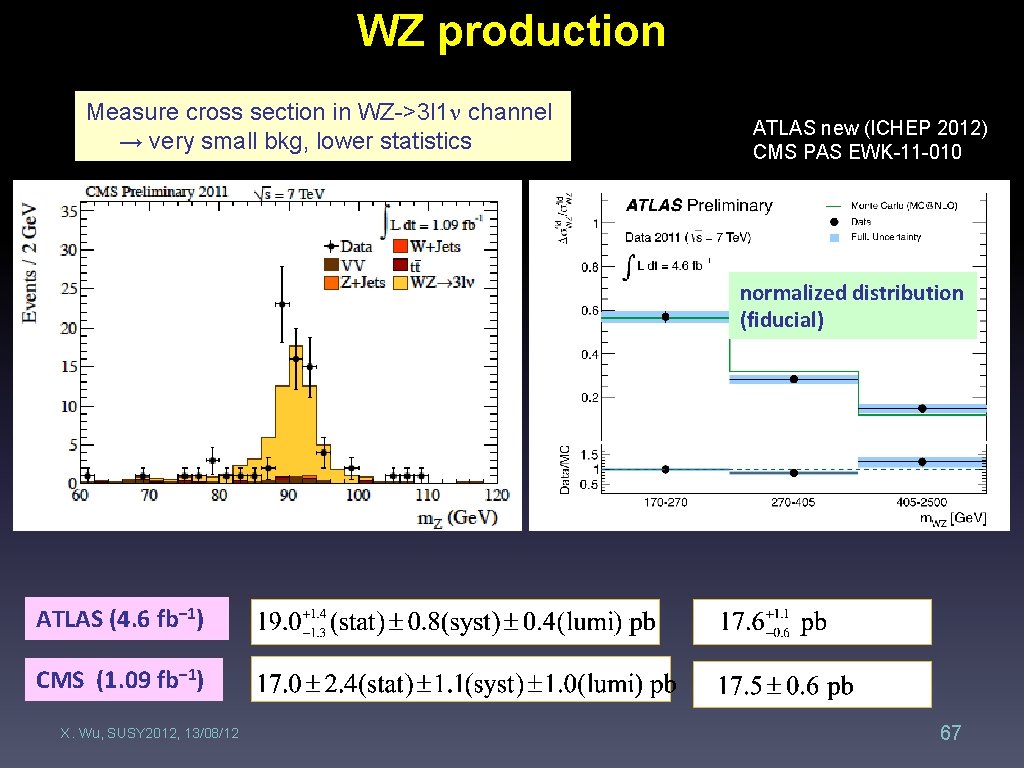
WZ production Measure cross section in WZ->3 l 1 channel → very small bkg, lower statistics ATLAS new (ICHEP 2012) CMS PAS EWK-11 -010 normalized distribution (fiducial) ATLAS (4. 6 fb− 1) CMS (1. 09 fb− 1) X. Wu, SUSY 2012, 13/08/12 67
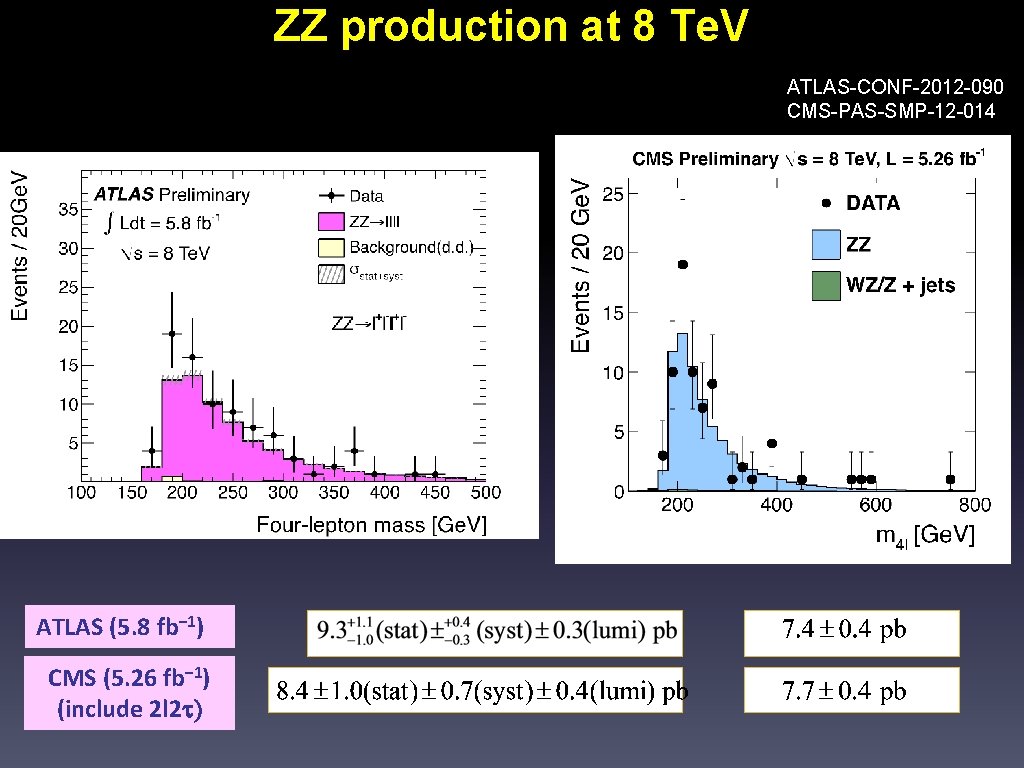
ZZ production at 8 Te. V ATLAS-CONF-2012 -090 CMS-PAS-SMP-12 -014 ATLAS (5. 8 fb− 1) CMS (5. 26 fb− 1) (include 2 l 2 t)
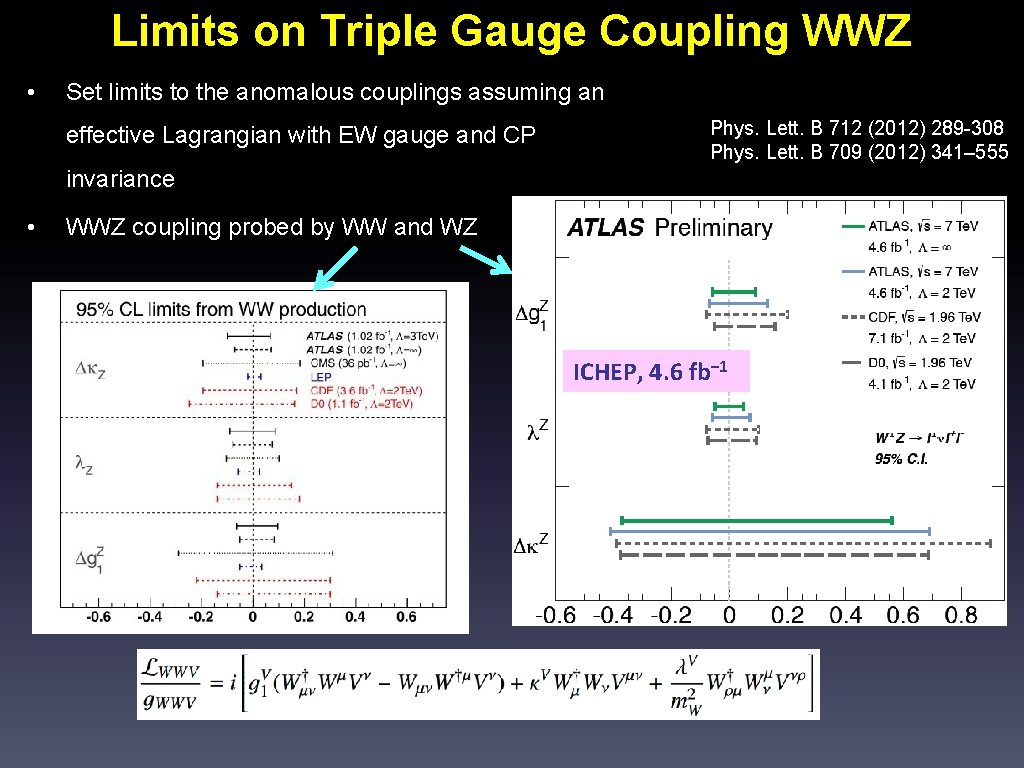
Limits on Triple Gauge Coupling WWZ • Set limits to the anomalous couplings assuming an effective Lagrangian with EW gauge and CP Phys. Lett. B 712 (2012) 289 -308 Phys. Lett. B 709 (2012) 341– 555 invariance • WWZ coupling probed by WW and WZ ICHEP, 4. 6 fb− 1
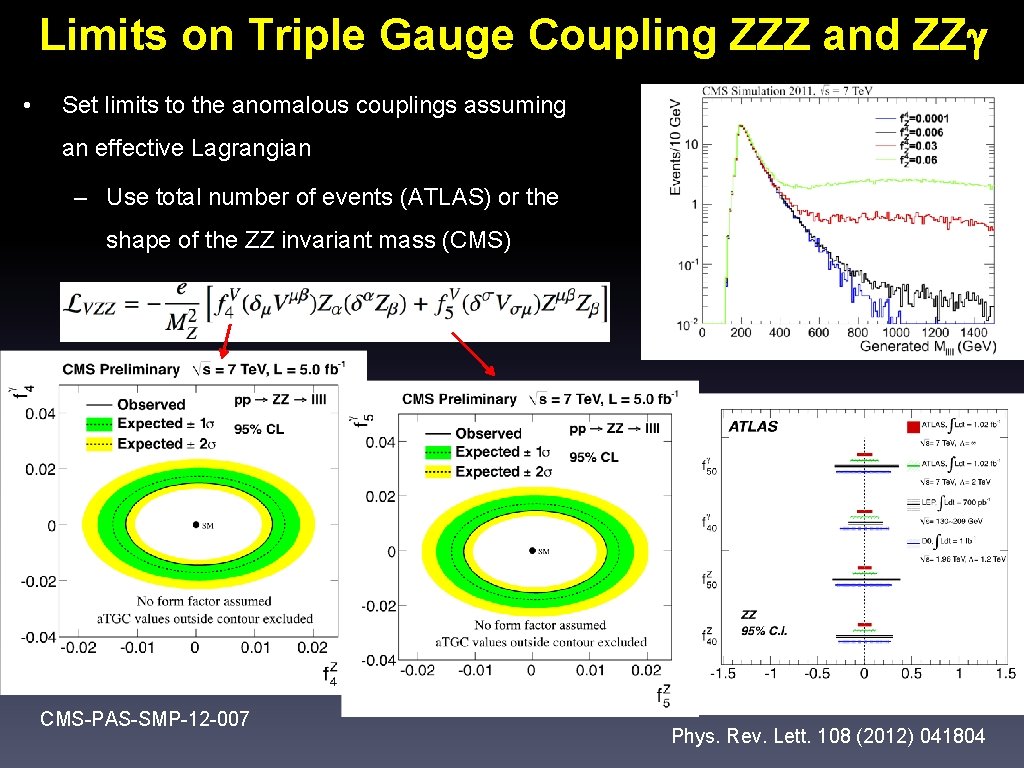
Limits on Triple Gauge Coupling ZZZ and ZZ • Set limits to the anomalous couplings assuming an effective Lagrangian – Use total number of events (ATLAS) or the shape of the ZZ invariant mass (CMS) CMS-PAS-SMP-12 -007 Phys. Rev. Lett. 108 (2012) 041804
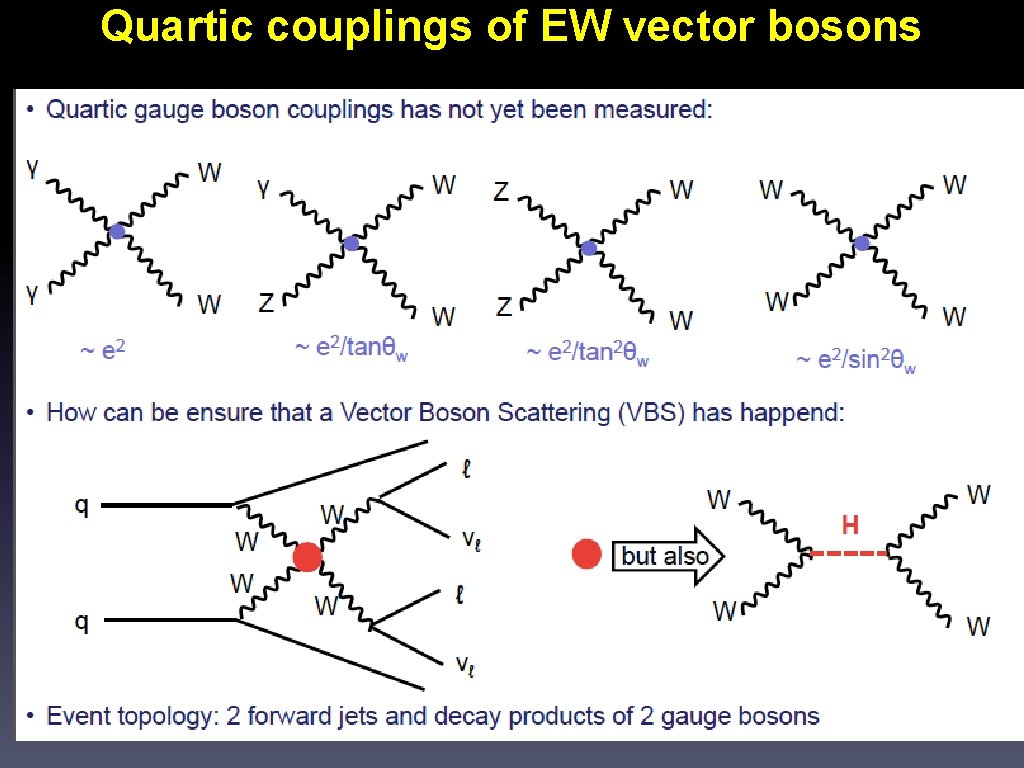
Quartic couplings of EW vector bosons
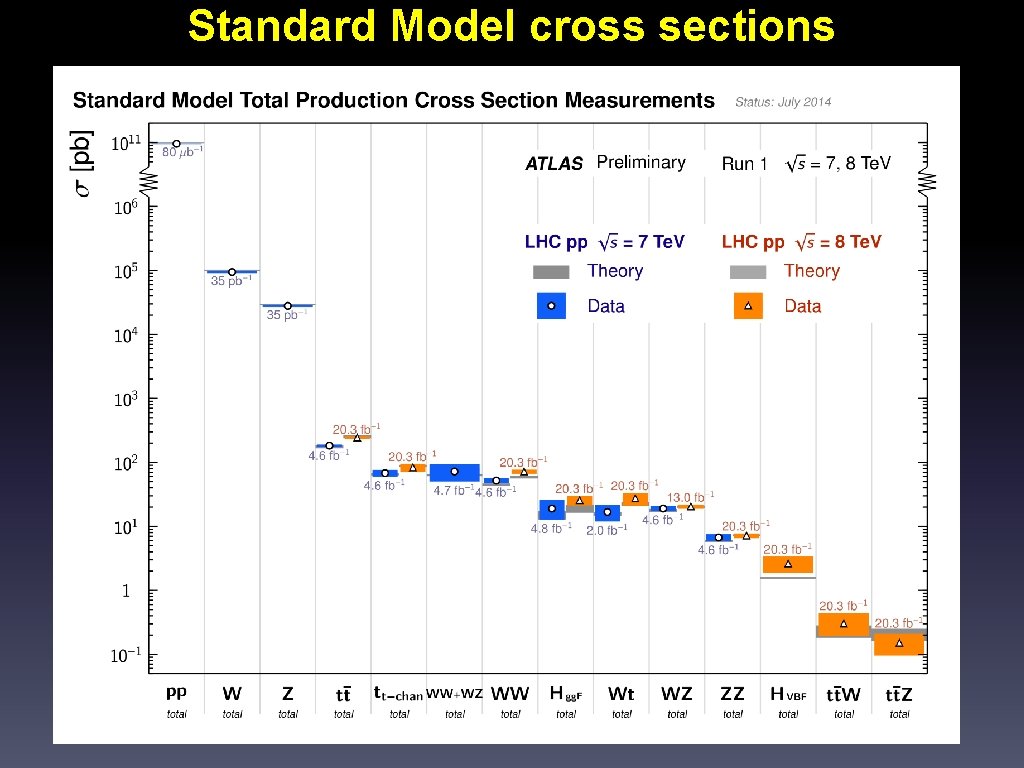
Standard Model cross sections
Hadron collider
Hadron calorimeter
Hadrons
Bellettini
Tevatron superjets
Hadron
Tevatron cdf
Hadron collider
Tevatron
Hamlet act iii scene ii
Why does it happen
University physics with modern physics fifteenth edition
Ib physics ia ideas
Auribus tenere lupum
The tragedy of julius caesar act 3
Vector iii
Neutral ligands
Pentium iii
Nelson-denny score chart
Vývojový test zrakového vnímání od frostigové
Title iii requirements
Hamlet summary act 3
Equivalent record form sample
Leo iii outlawed crucifixes as idolatry.
Microaggressions
Nic iii
Bsid-iii-nl
Aluminum and iron iii oxide balanced equation
7 ano iii bimestre aula 1
Bazel iii
Clasificacion de hinchey
Emil nolde natura morta con maschere
Kara walker: darkytown rebellion, 2001 stadia ii
Motywy biblijne w dziadach cz 3
Criterios de roma iii
3 condicional
George nichols iii
Hcps iii
Hikayat pahang
Ulcera forrest classificazione
Iron(iii) chloride (aq) + cesium phosphate (aq)
George washington and john adams venn diagram
Group iii elements
Petra iii status
Interreg iii b
V chwyt leopolda
Hbcu title iii administrators
Kahalagahan ng pag aaral sa pananaliksik kahulugan
Triamminetrichlorocobalt(iii)
In act 3 mercutio and benvolio get
Iii domingo de cuaresma ciclo c
Solvency 2 pillar 3
Sals lighting system
Thora seal instructions
Reverend hale the crucible
Mrp i mrp ii
Cadses
Telpas headphones
Divina commedia canto 30 inferno
Rozbior
Cuarto plano de hodge
Innocenzo iii
Iii domenica di avvento anno c
Mrp mrpii
Hltpat001
Motet definition
Wadah yang dapat mencegah menembusnya udara/gas adalah
English level iii
Hamlet act iv scene vii
Dario iii
King george iii
Mrp rendszer
Wppsi iii instrucciones