Nonlinear Spectral Analysis in Aeroacoustics Dr K Srinivasan
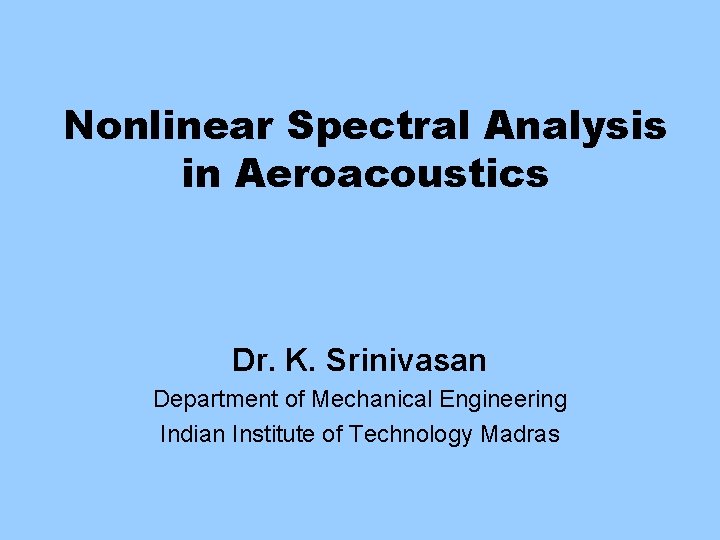
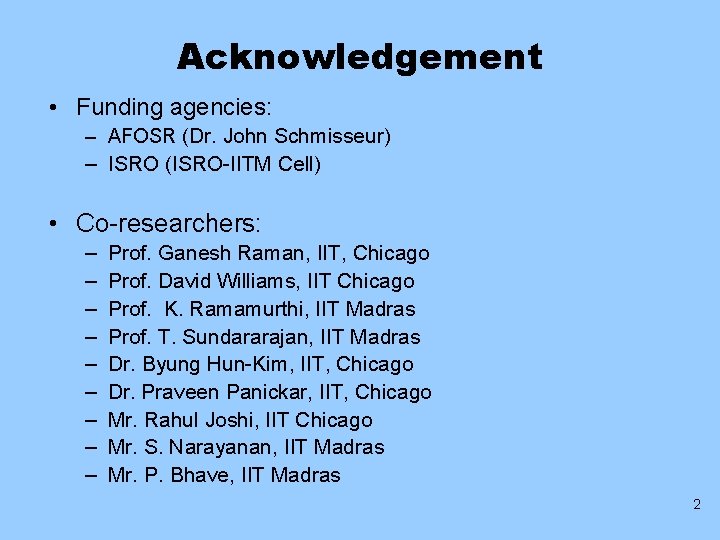
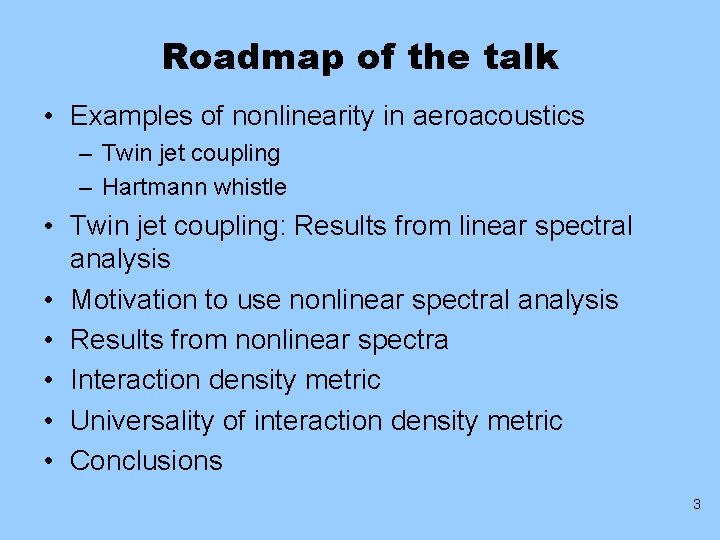
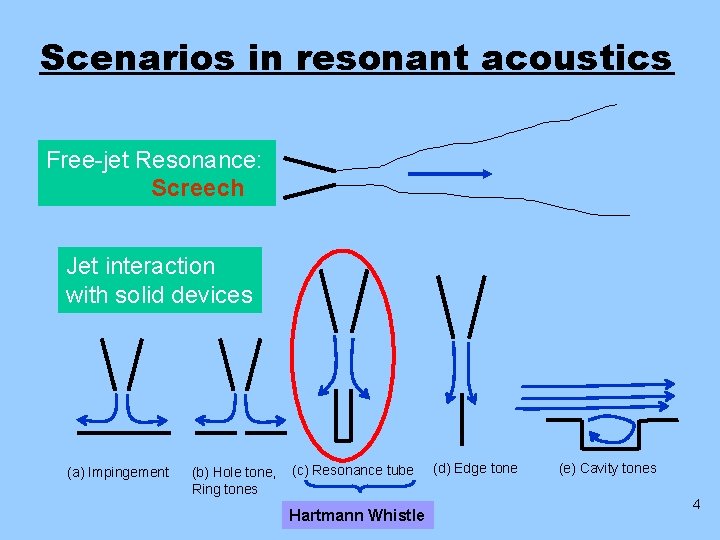
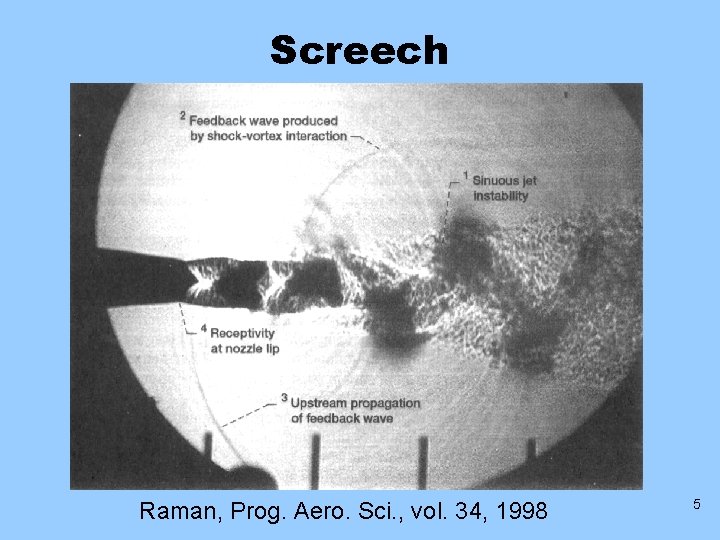
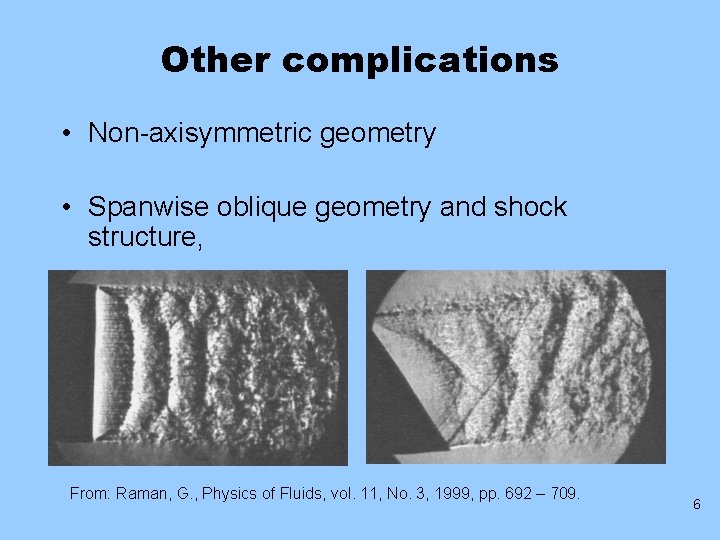
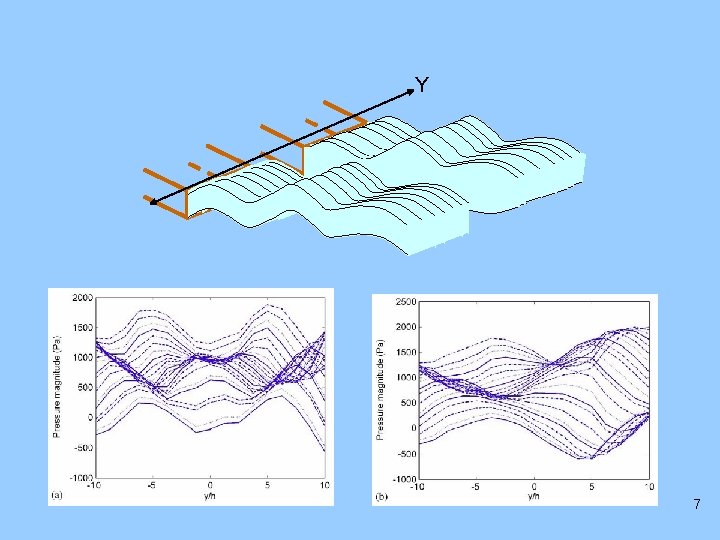
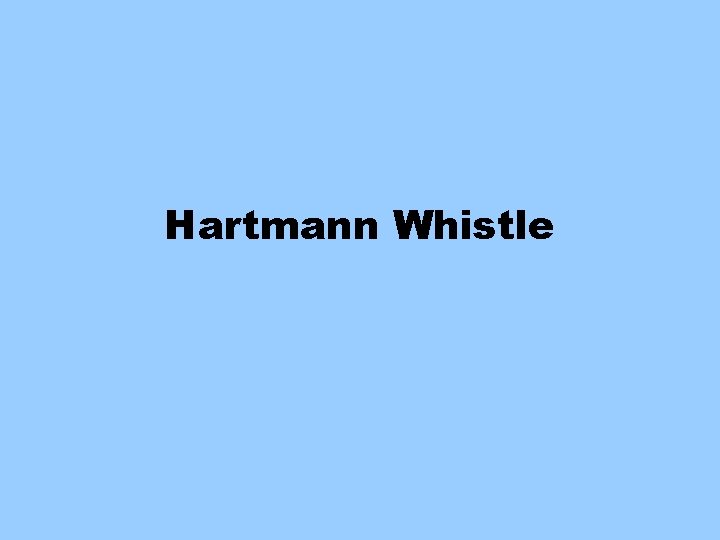
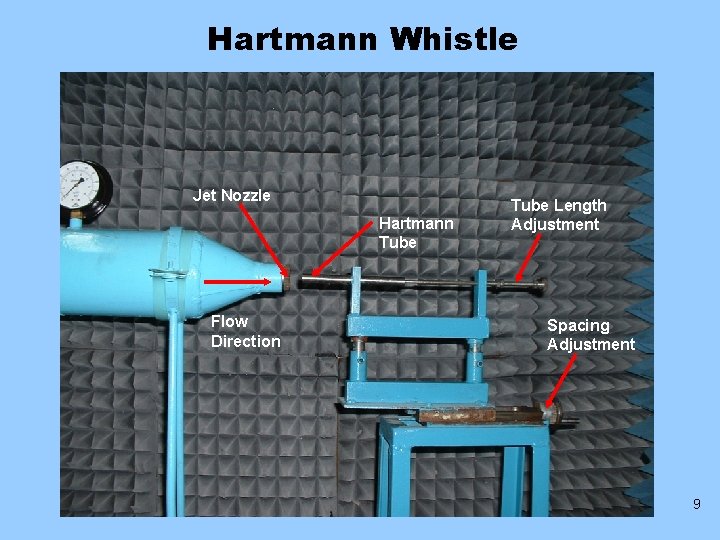
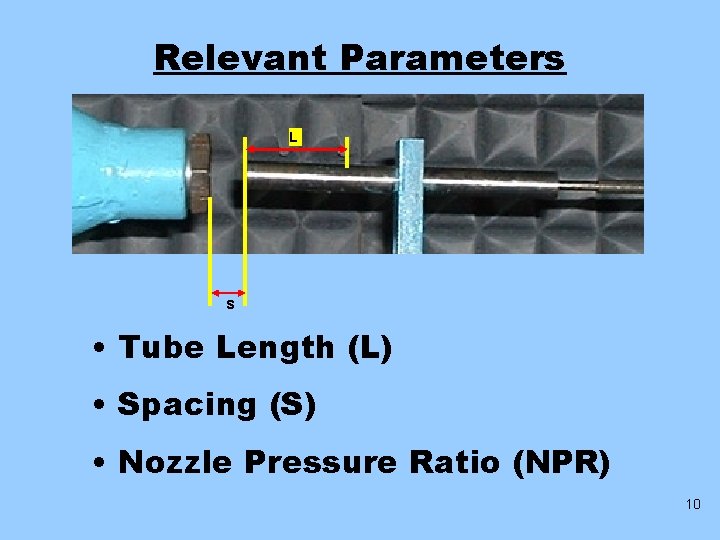
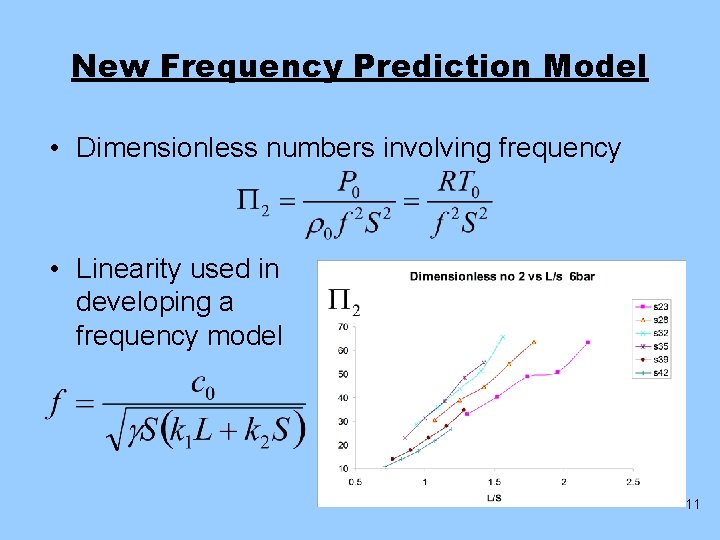
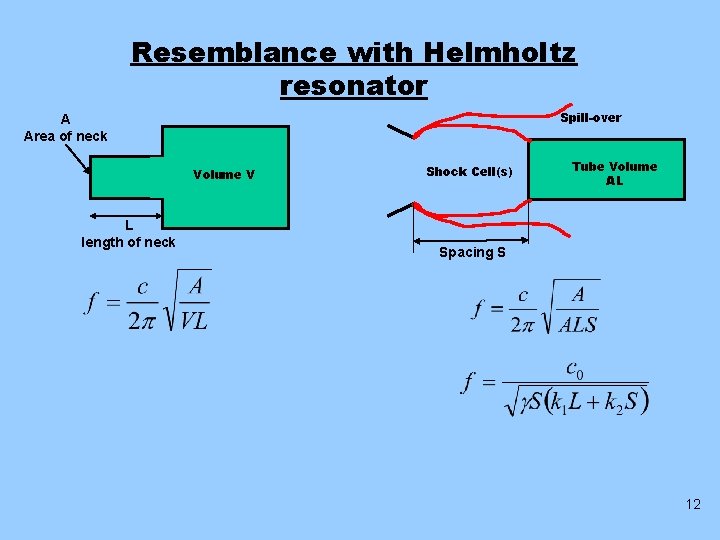
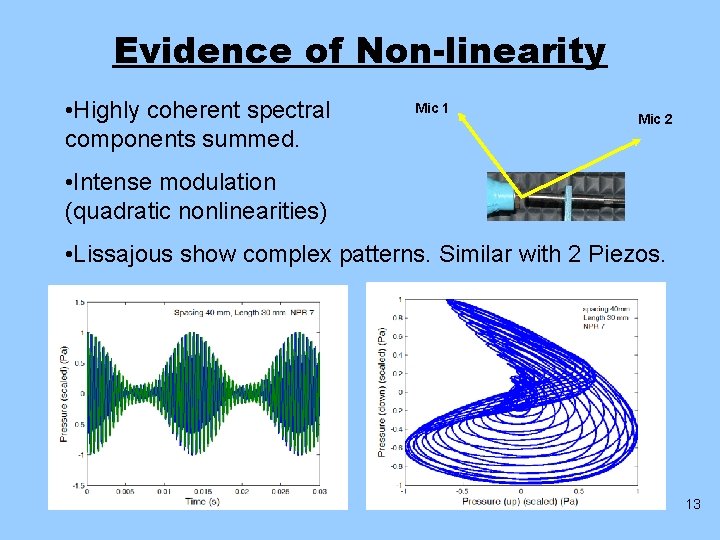
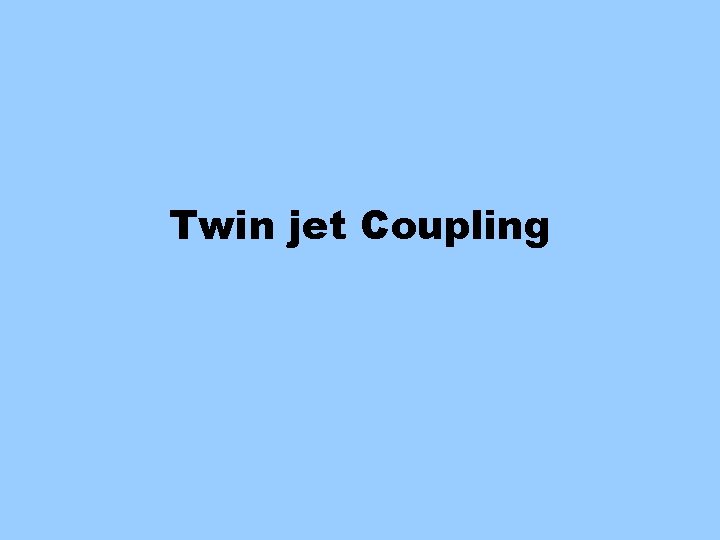
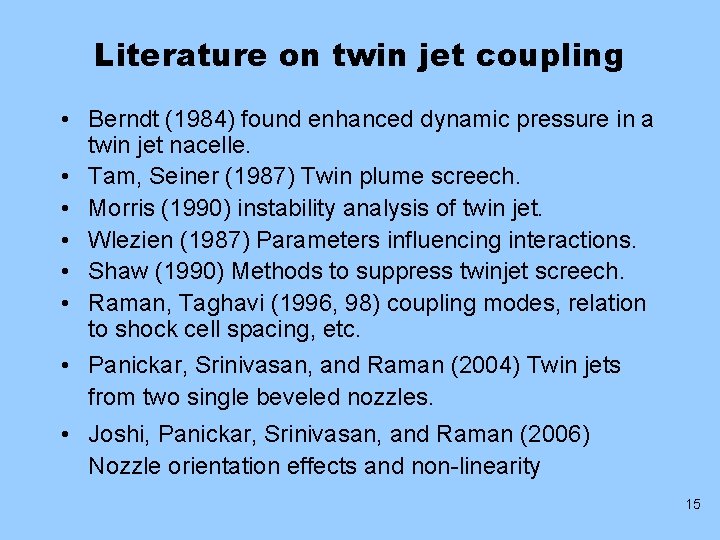
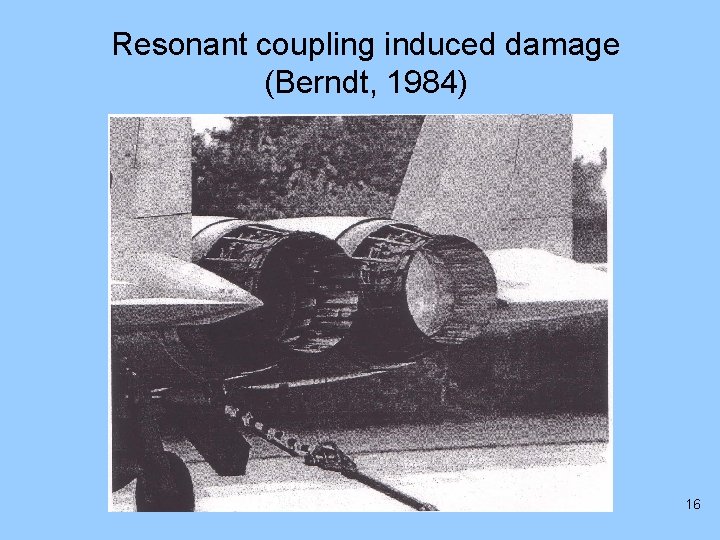
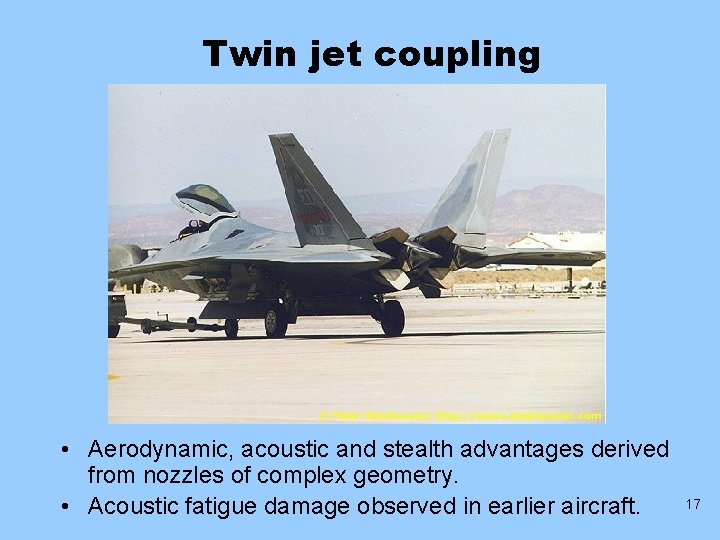
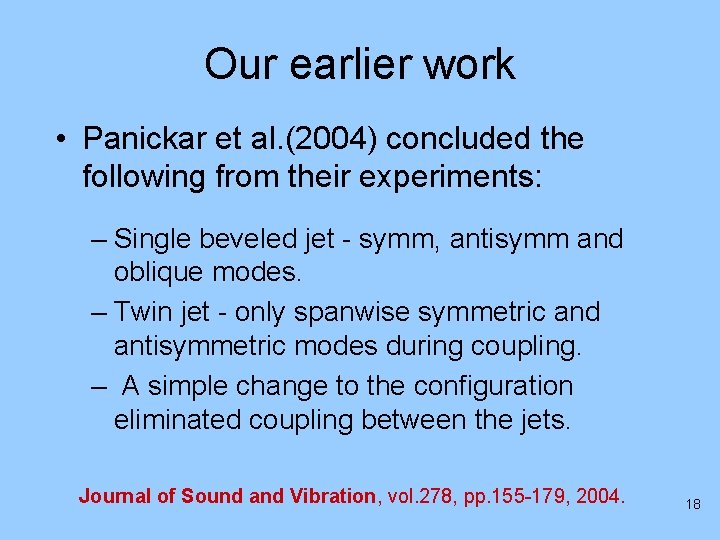
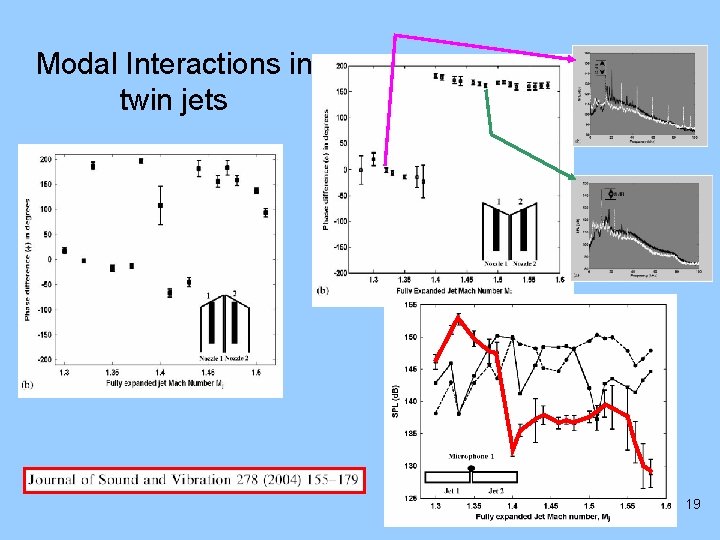
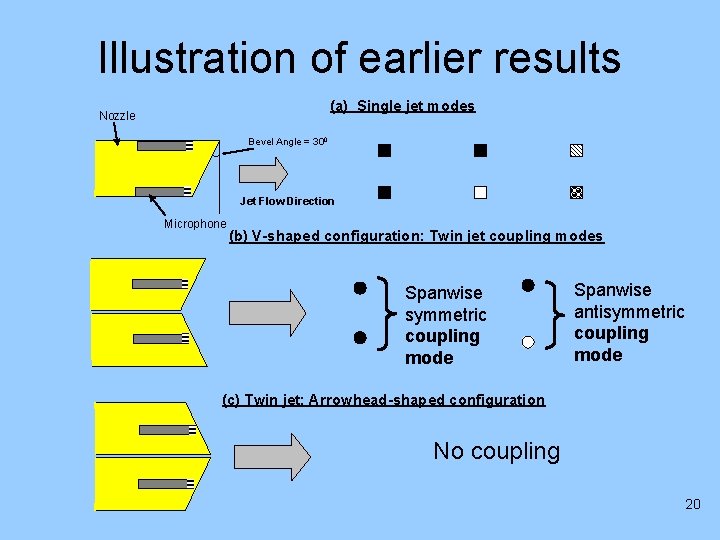
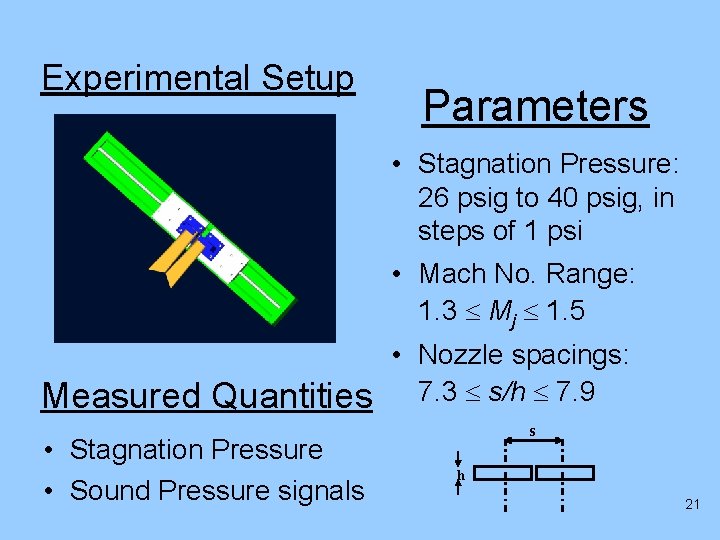
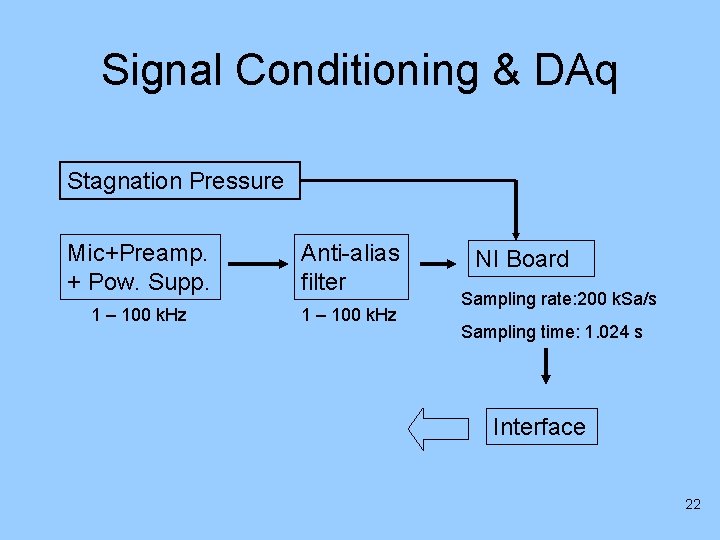
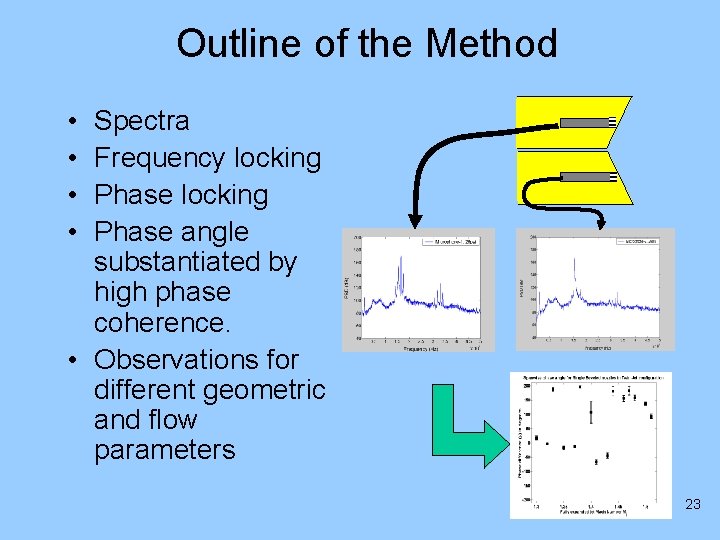
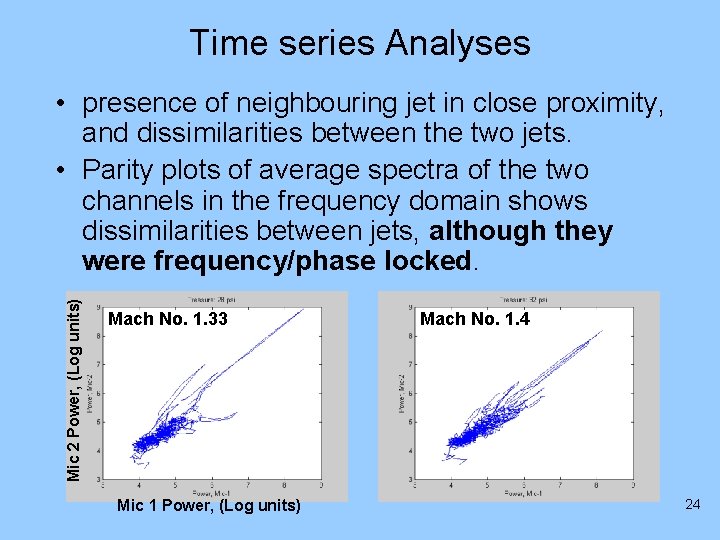
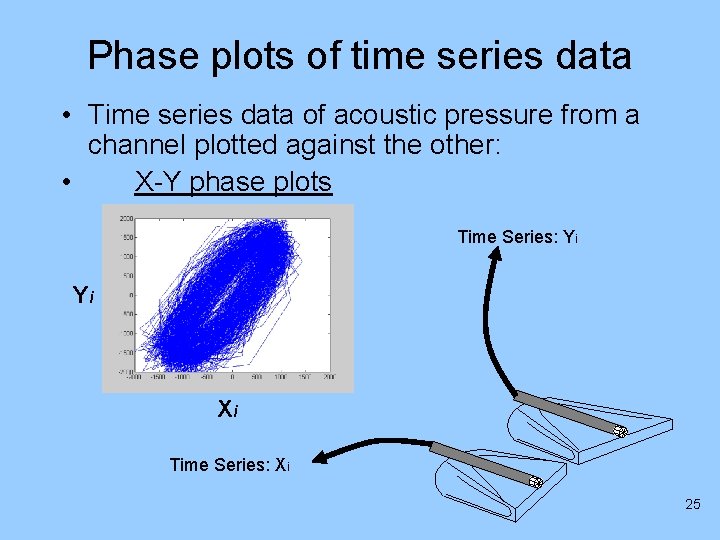
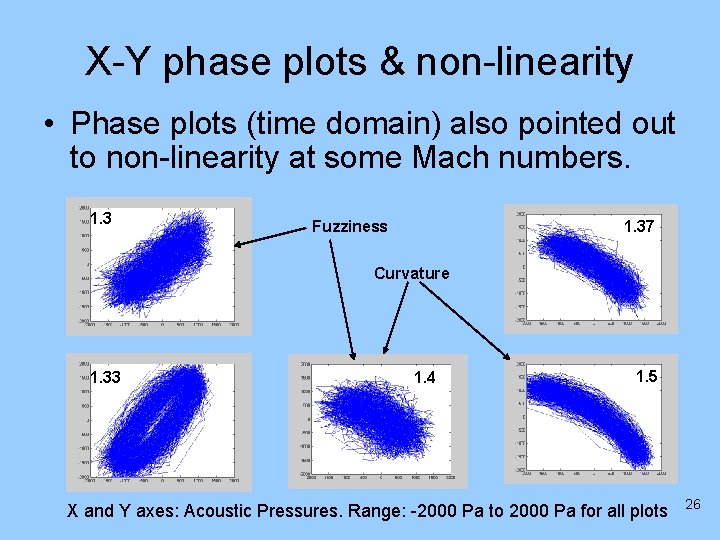
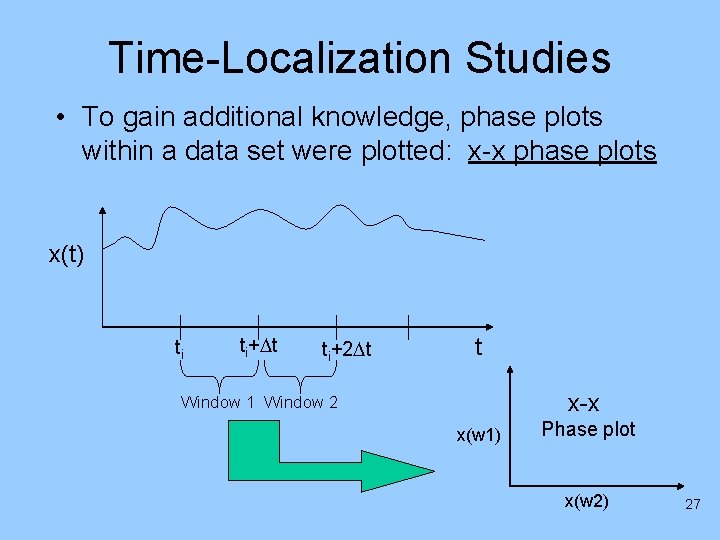
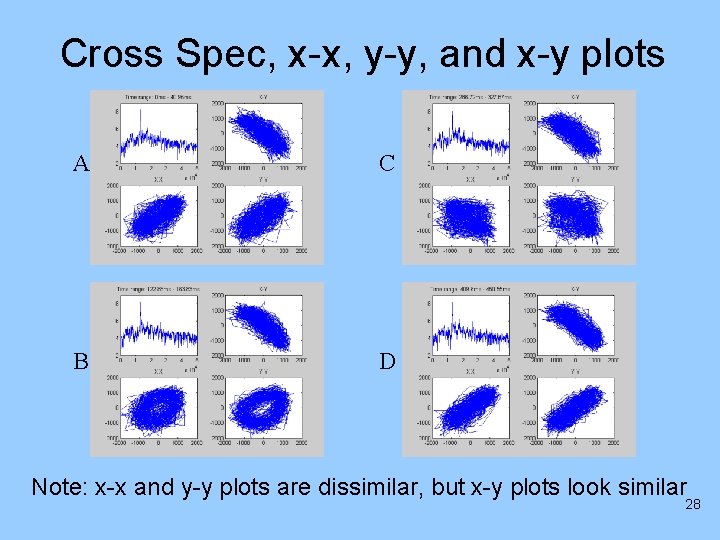
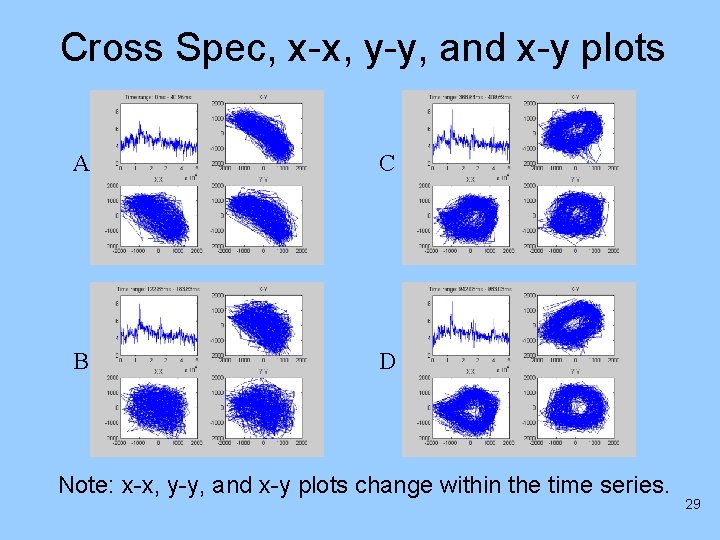
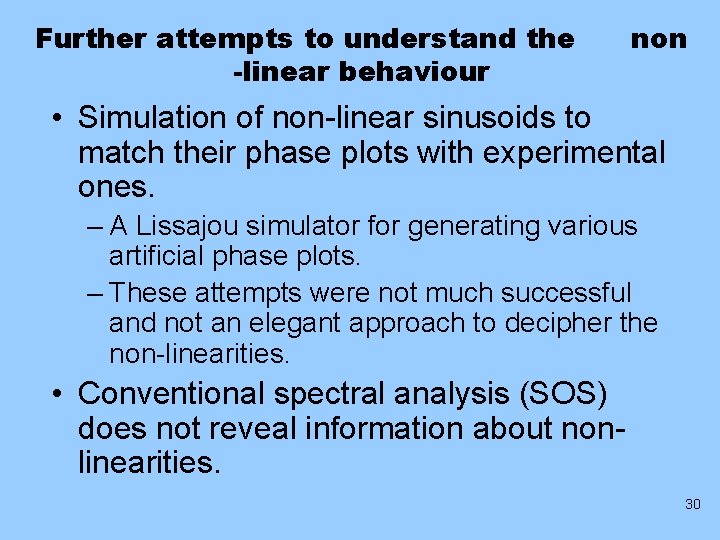
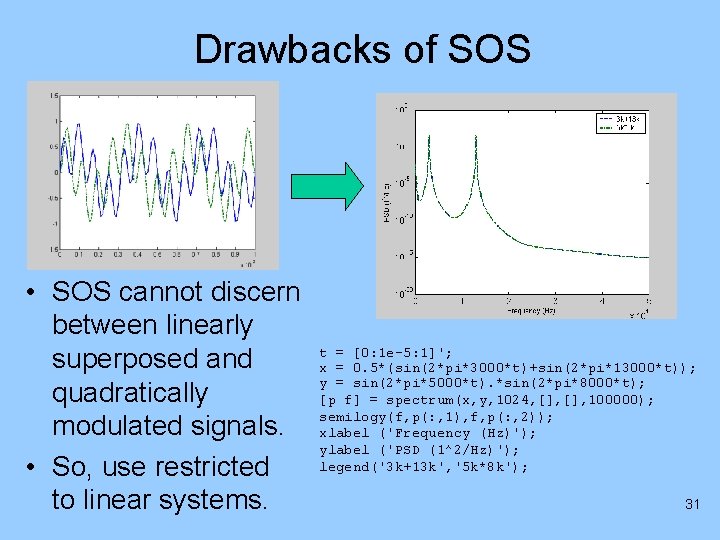
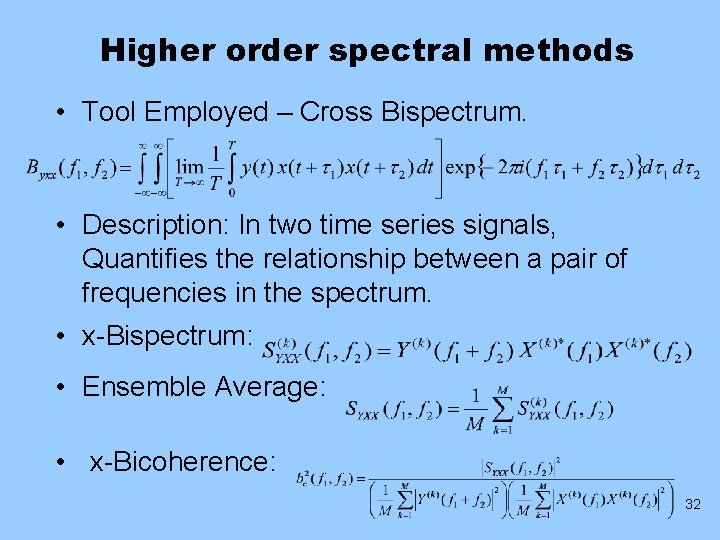
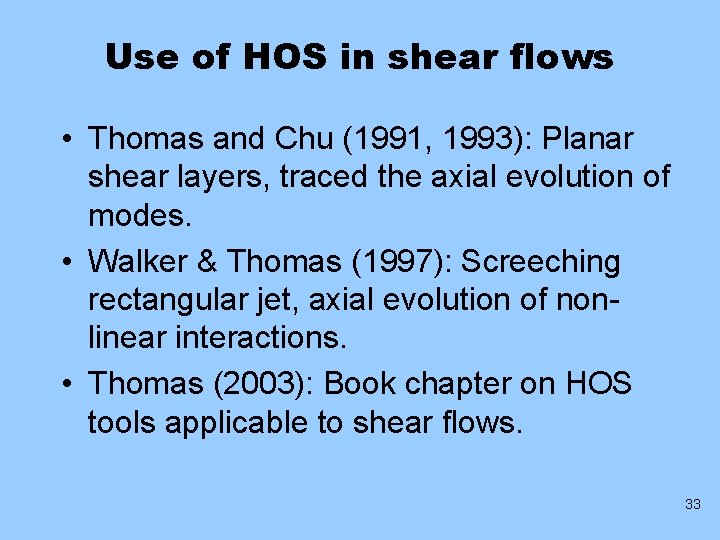
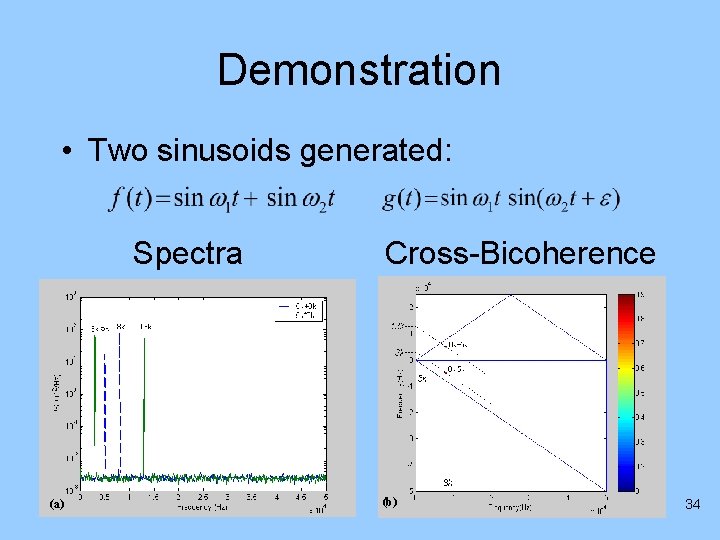
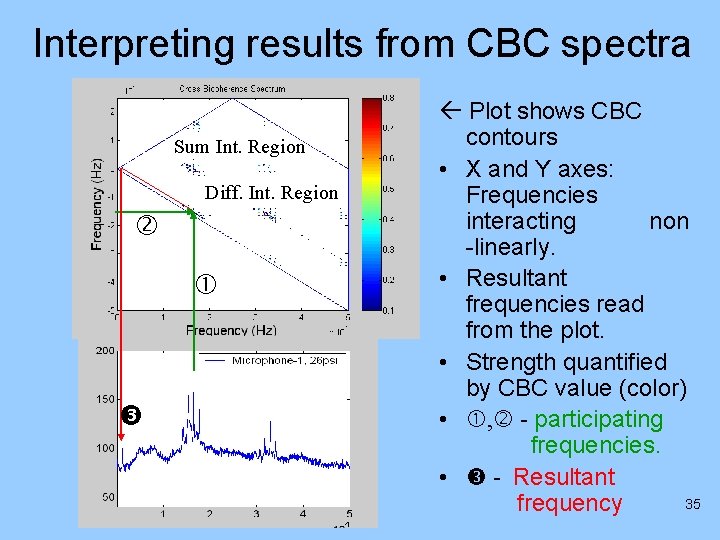
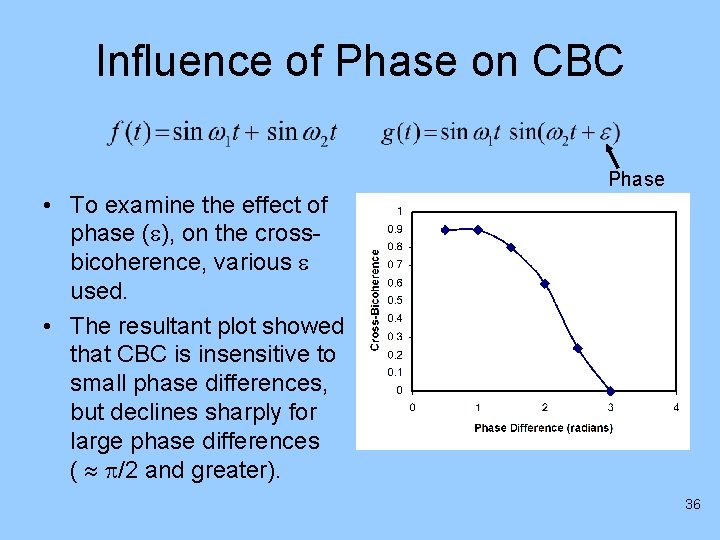
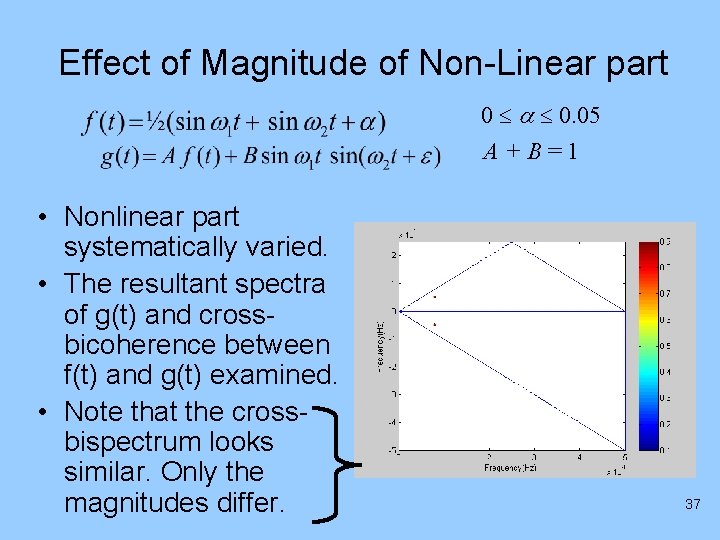
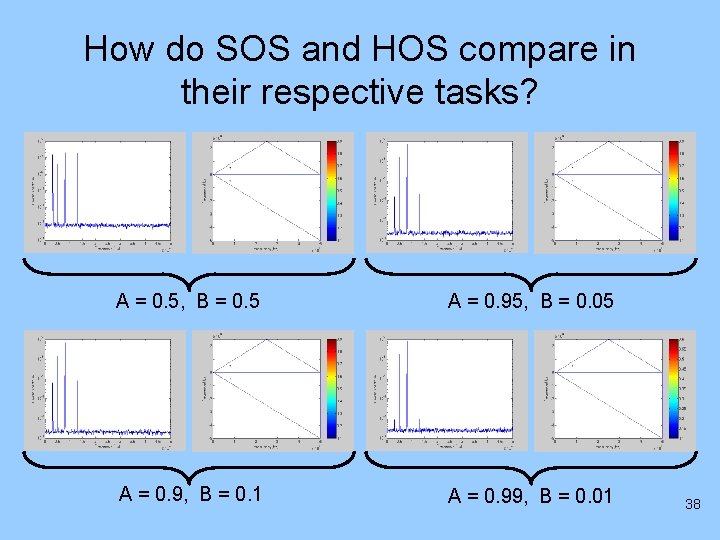
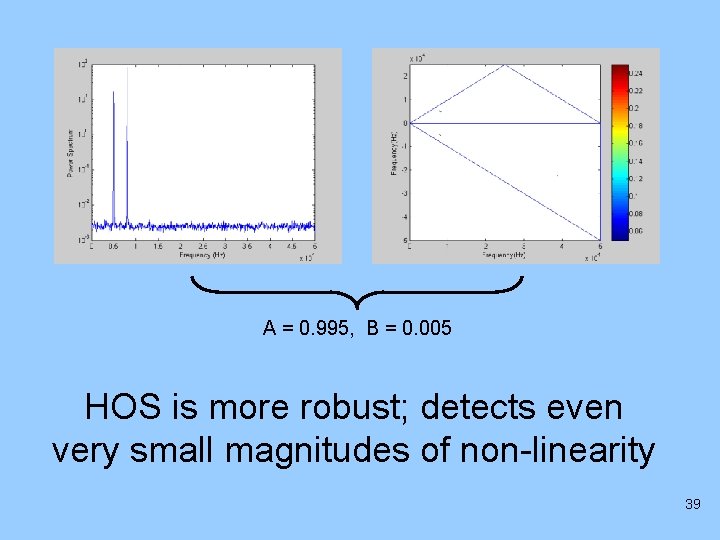
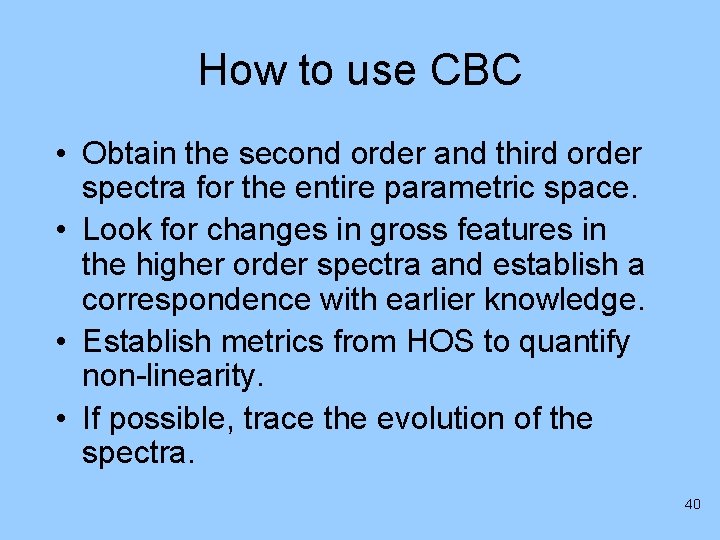
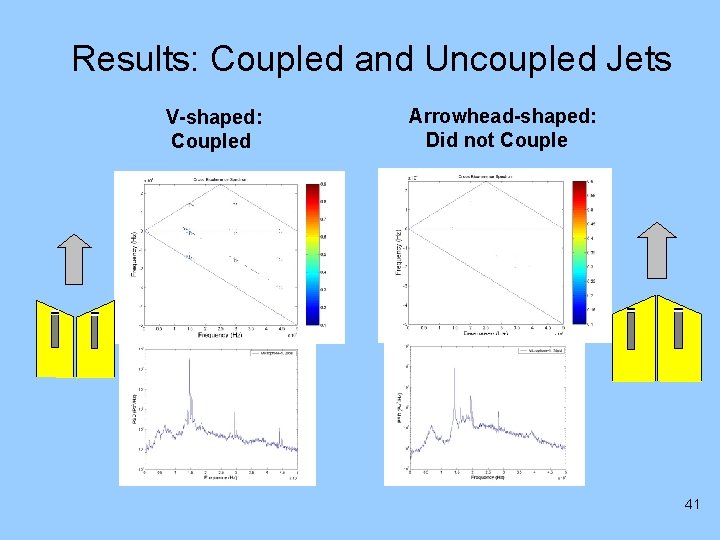
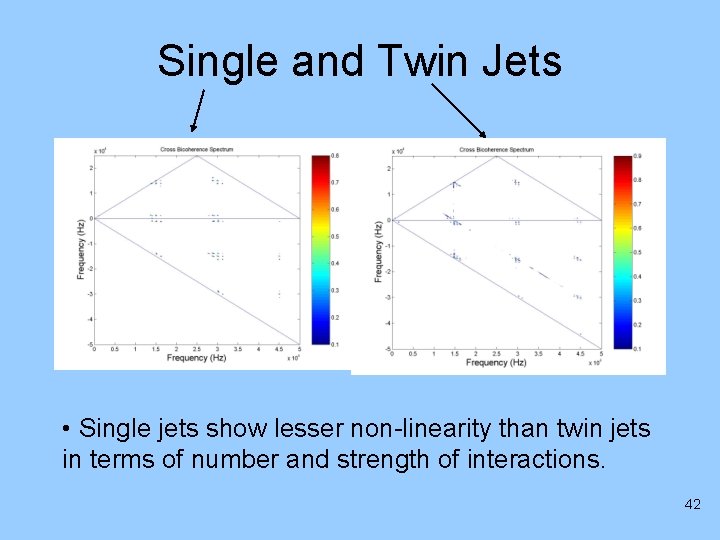
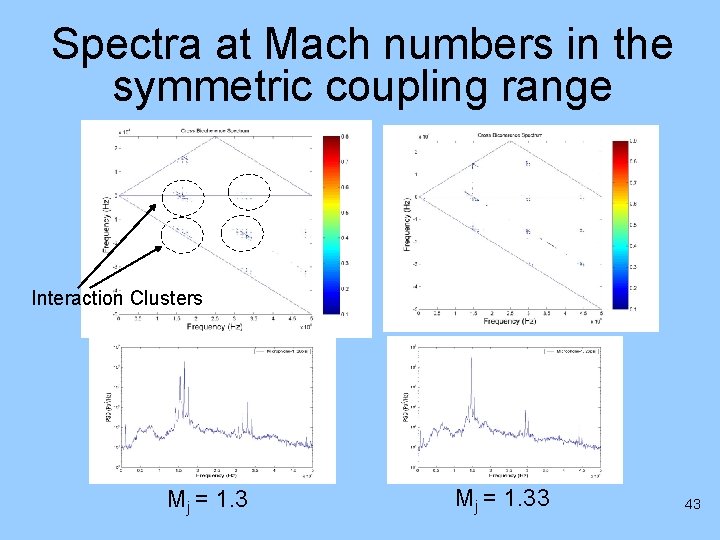
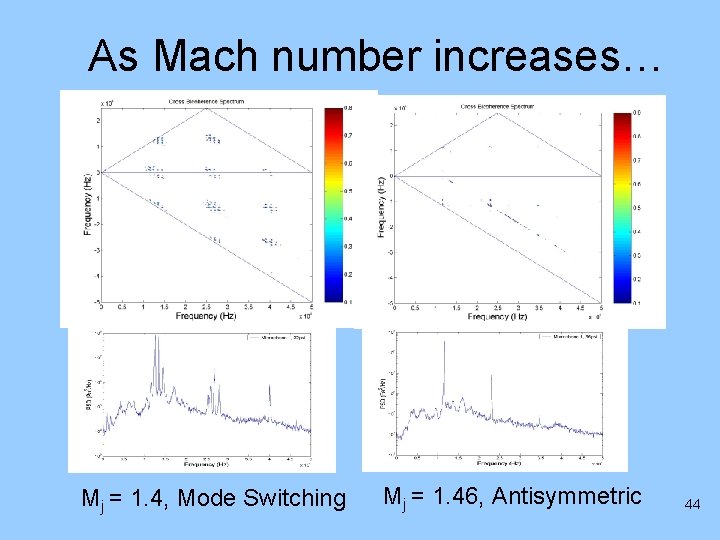
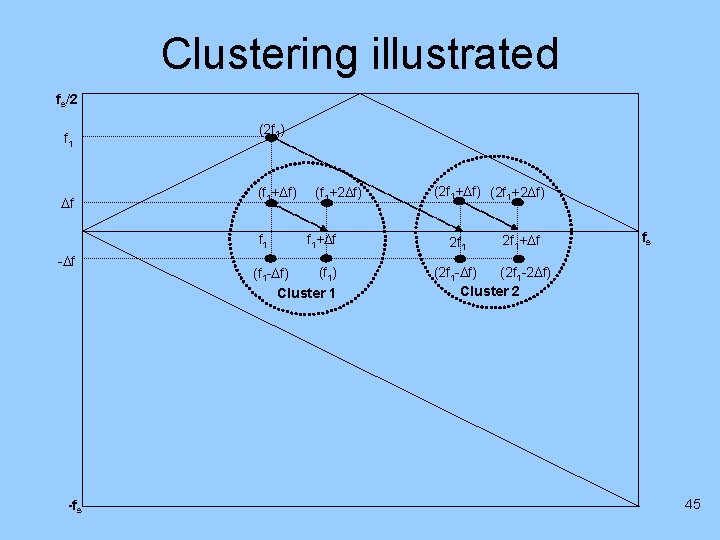
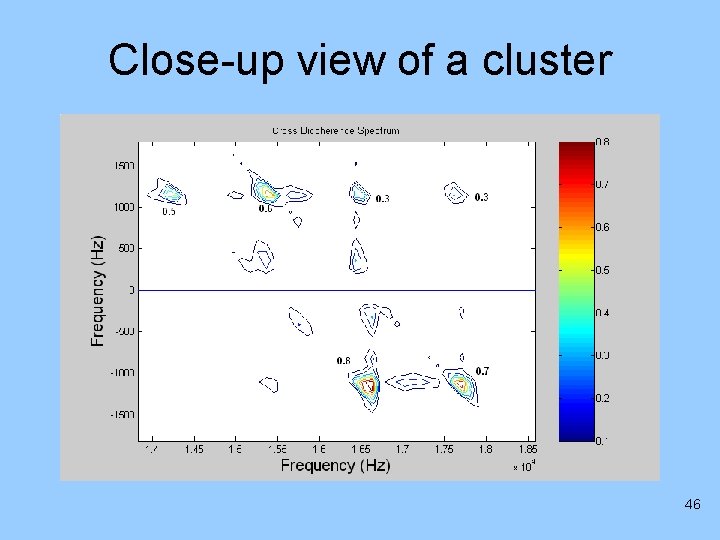
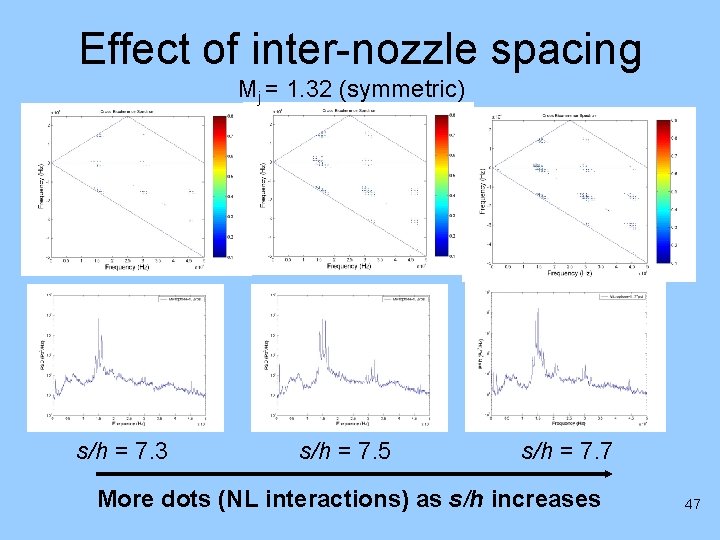
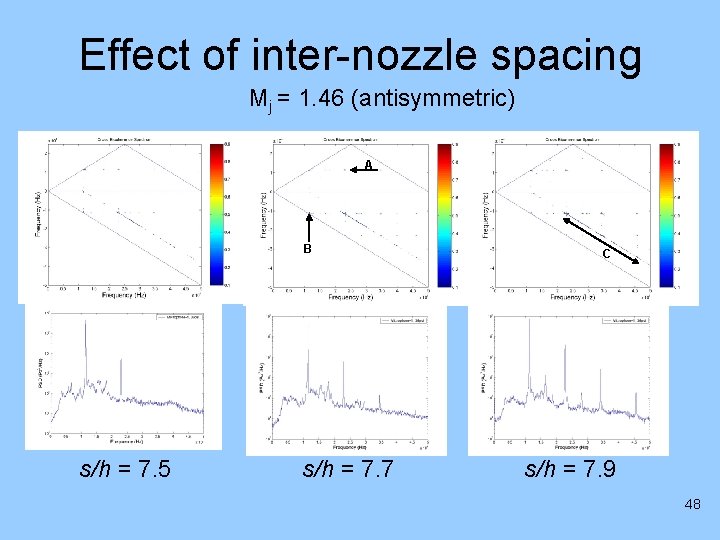
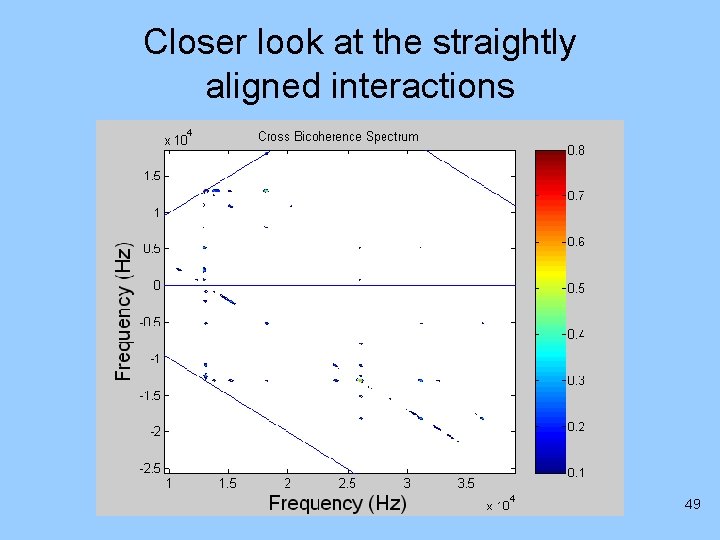
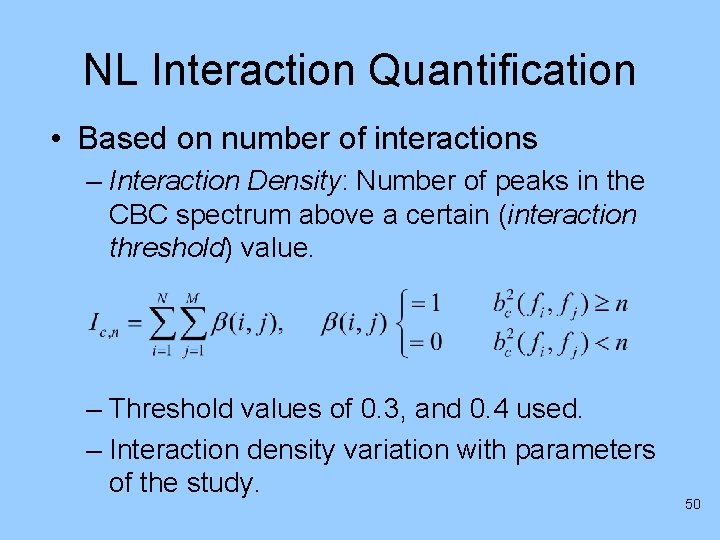
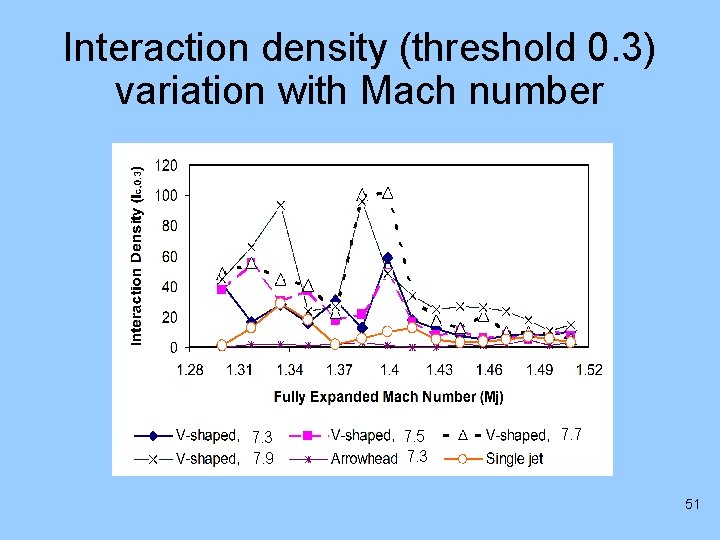
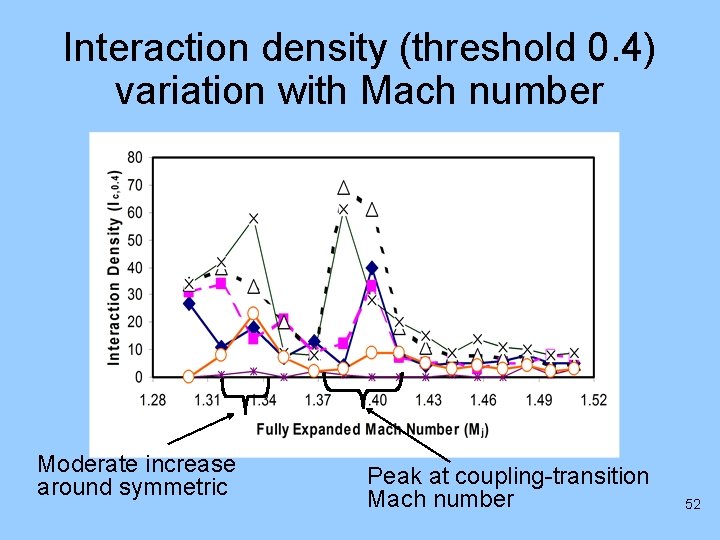
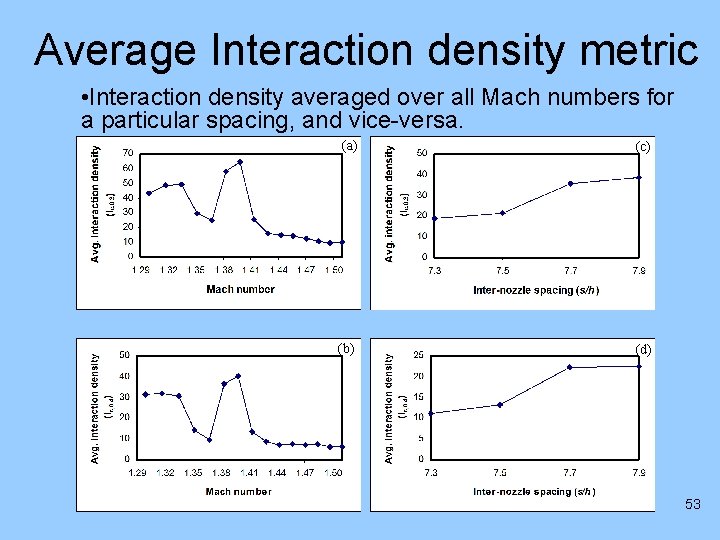
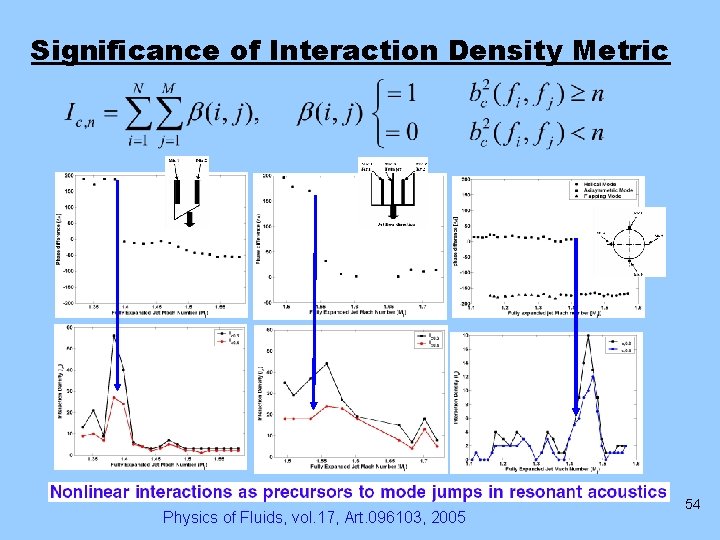
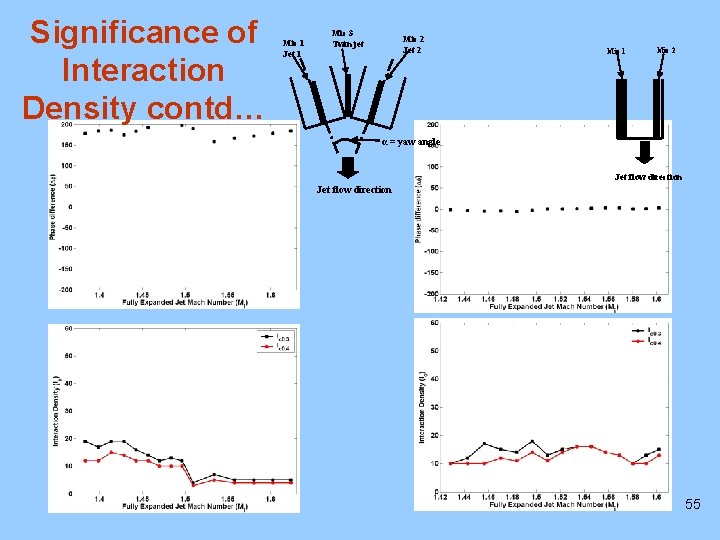
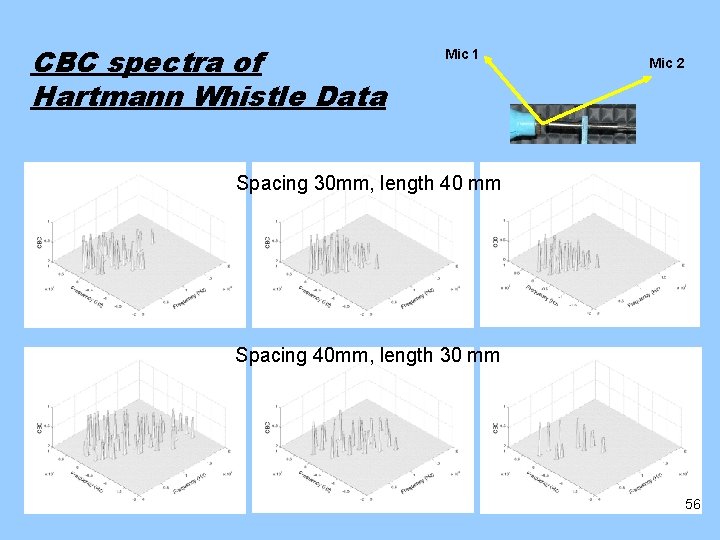
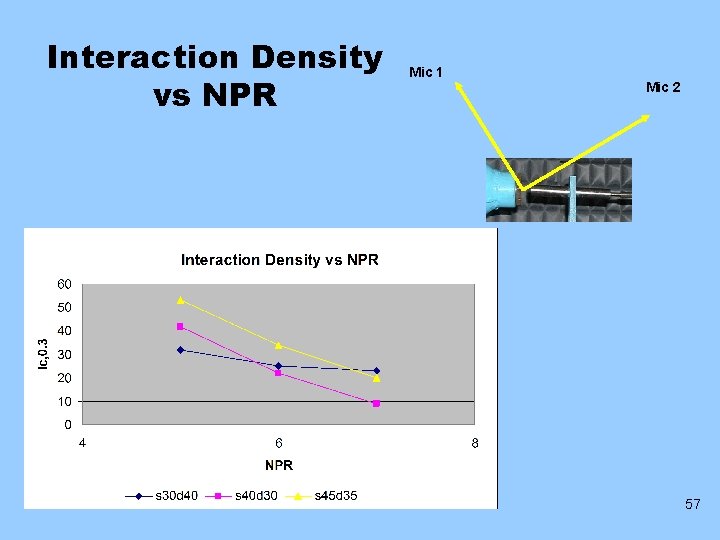
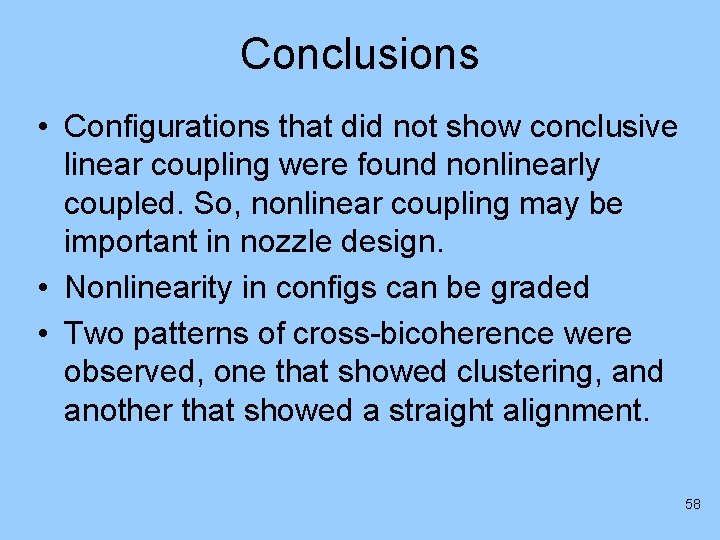
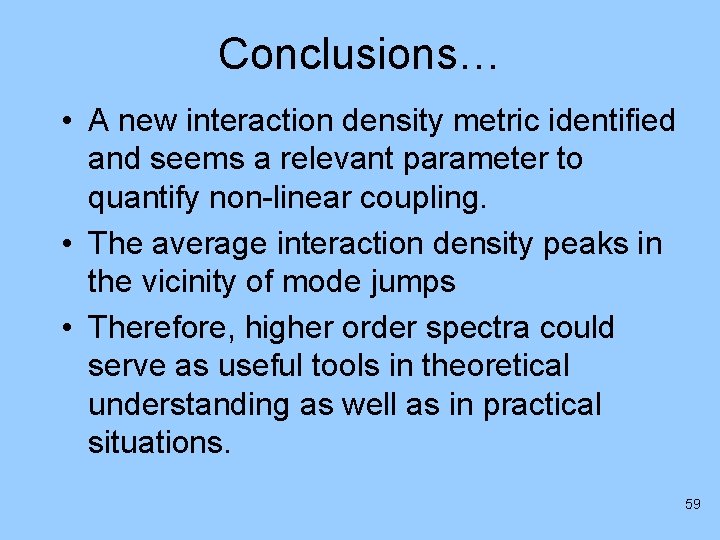
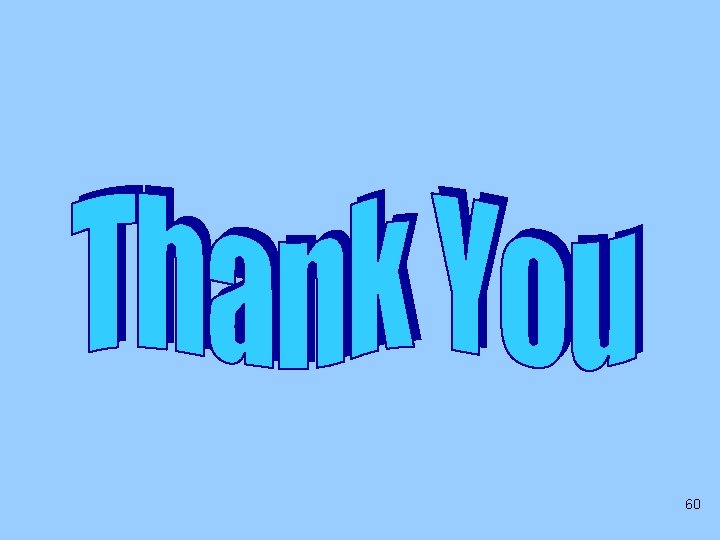
- Slides: 60
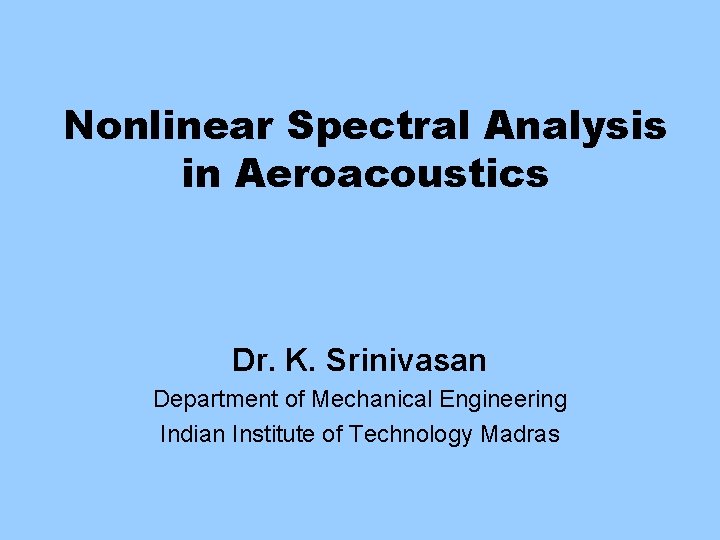
Nonlinear Spectral Analysis in Aeroacoustics Dr. K. Srinivasan Department of Mechanical Engineering Indian Institute of Technology Madras
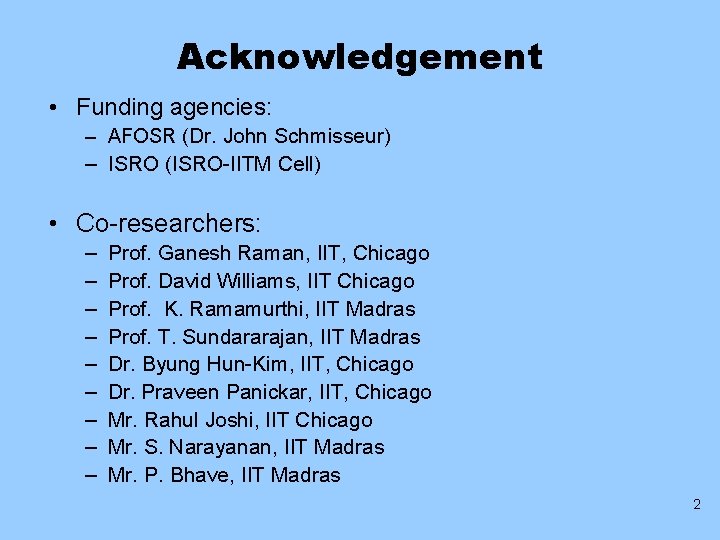
Acknowledgement • Funding agencies: – AFOSR (Dr. John Schmisseur) – ISRO (ISRO-IITM Cell) • Co-researchers: – – – – – Prof. Ganesh Raman, IIT, Chicago Prof. David Williams, IIT Chicago Prof. K. Ramamurthi, IIT Madras Prof. T. Sundararajan, IIT Madras Dr. Byung Hun-Kim, IIT, Chicago Dr. Praveen Panickar, IIT, Chicago Mr. Rahul Joshi, IIT Chicago Mr. S. Narayanan, IIT Madras Mr. P. Bhave, IIT Madras 2
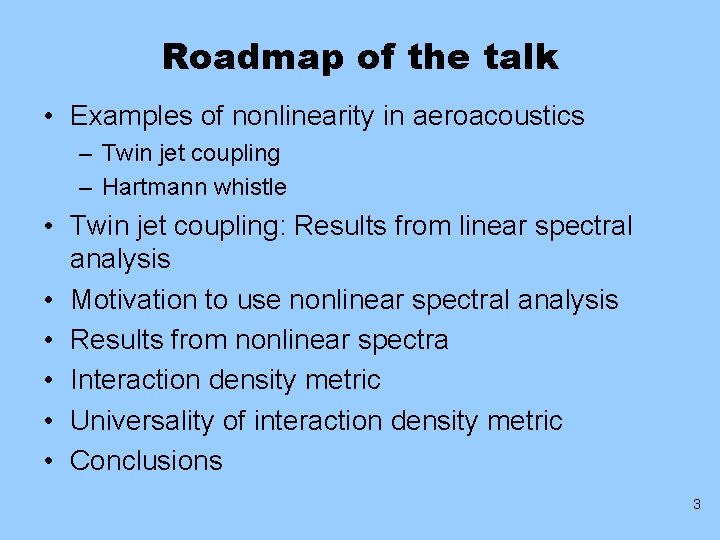
Roadmap of the talk • Examples of nonlinearity in aeroacoustics – Twin jet coupling – Hartmann whistle • Twin jet coupling: Results from linear spectral analysis • Motivation to use nonlinear spectral analysis • Results from nonlinear spectra • Interaction density metric • Universality of interaction density metric • Conclusions 3
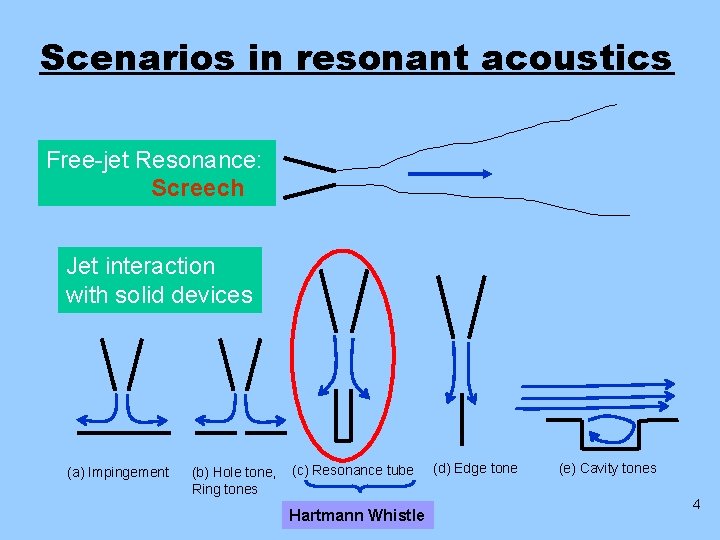
Scenarios in resonant acoustics Free-jet Resonance: Screech Jet interaction with solid devices (a) Impingement (b) Hole tone, Ring tones (c) Resonance tube Hartmann Whistle (d) Edge tone (e) Cavity tones 4
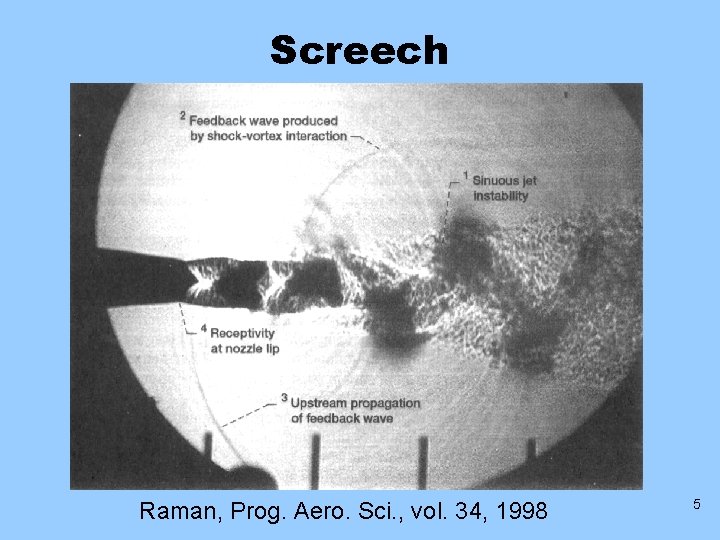
Screech Raman, Prog. Aero. Sci. , vol. 34, 1998 5
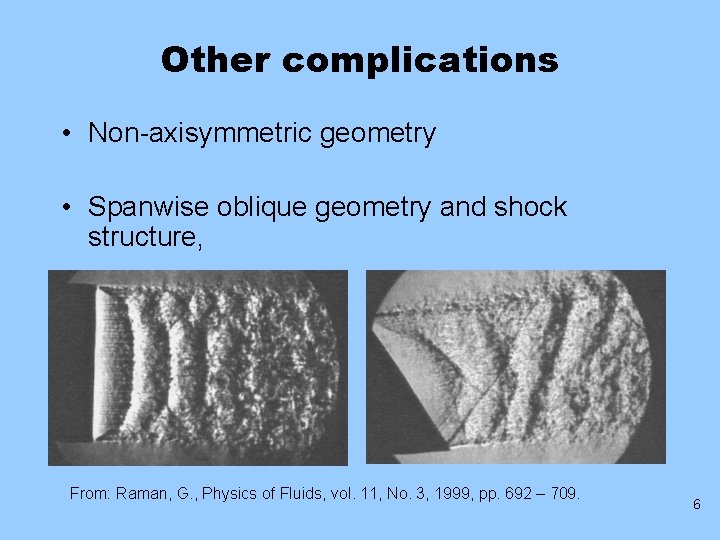
Other complications • Non-axisymmetric geometry • Spanwise oblique geometry and shock structure, From: Raman, G. , Physics of Fluids, vol. 11, No. 3, 1999, pp. 692 – 709. 6
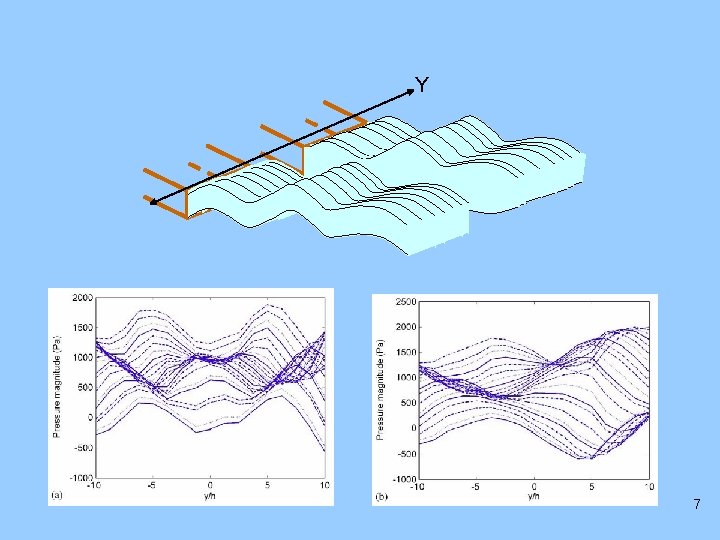
Y 7
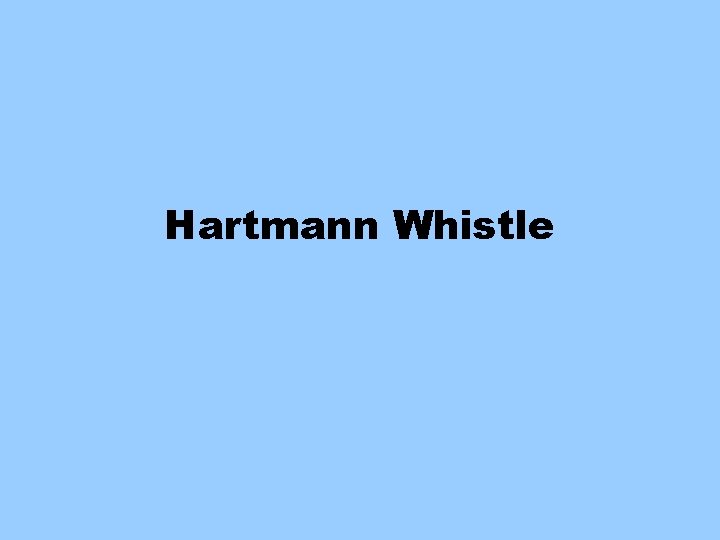
Hartmann Whistle
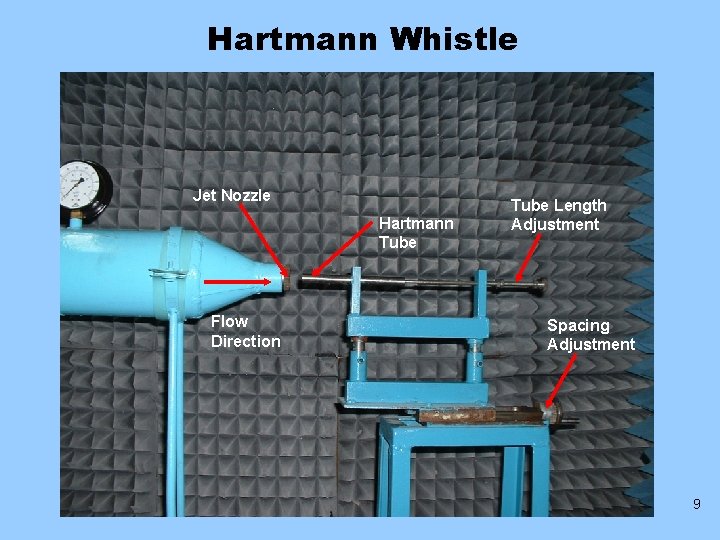
Hartmann Whistle Jet Nozzle Hartmann Tube Flow Direction Tube Length Adjustment Spacing Adjustment 9
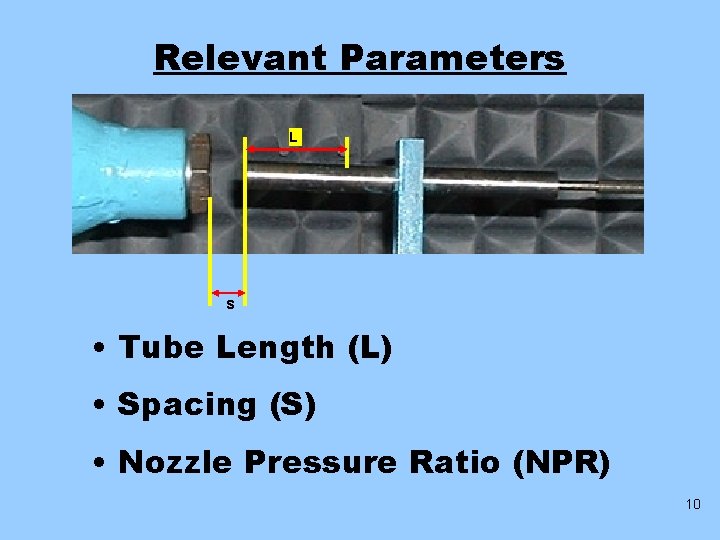
Relevant Parameters L s • Tube Length (L) • Spacing (S) • Nozzle Pressure Ratio (NPR) 10
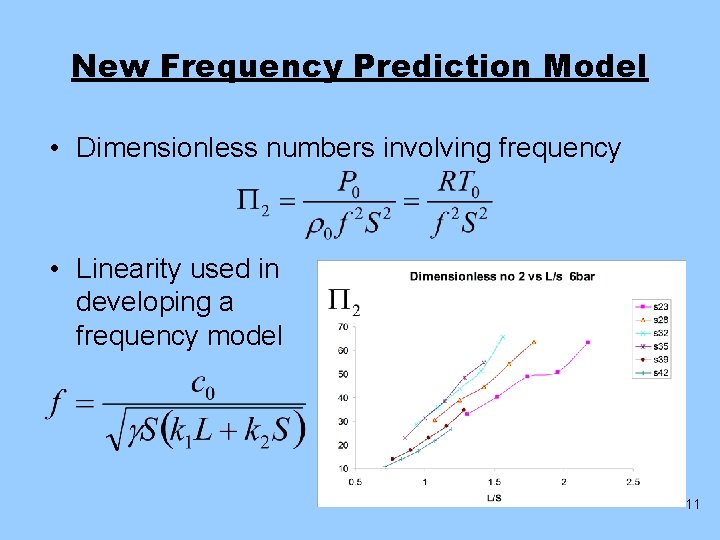
New Frequency Prediction Model • Dimensionless numbers involving frequency • Linearity used in developing a frequency model 11
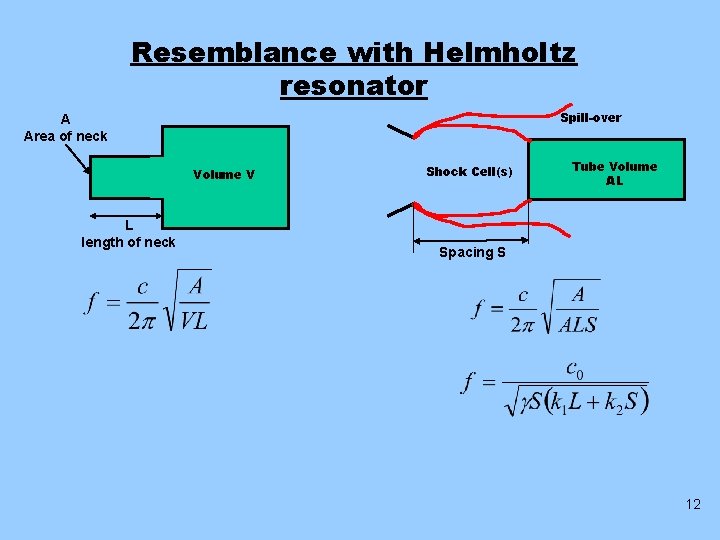
Resemblance with Helmholtz resonator Spill-over A Area of neck Volume V L length of neck Shock Cell(s) Tube Volume AL Spacing S 12
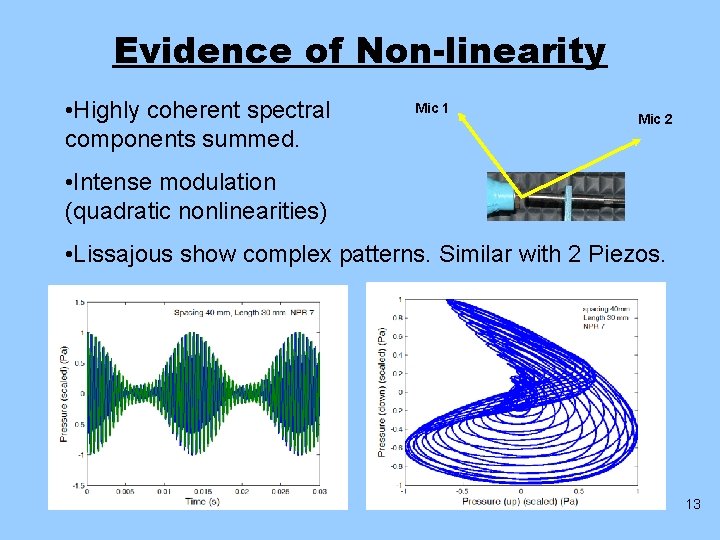
Evidence of Non-linearity • Highly coherent spectral components summed. Mic 1 Mic 2 • Intense modulation (quadratic nonlinearities) • Lissajous show complex patterns. Similar with 2 Piezos. 13
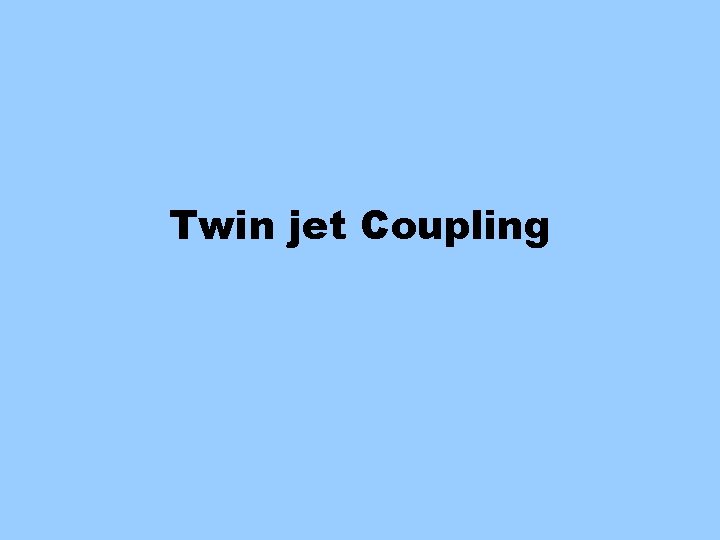
Twin jet Coupling
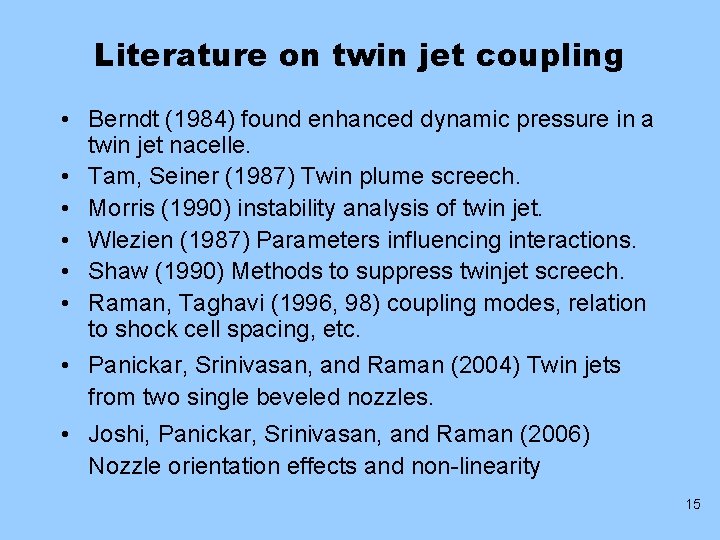
Literature on twin jet coupling • Berndt (1984) found enhanced dynamic pressure in a twin jet nacelle. • Tam, Seiner (1987) Twin plume screech. • Morris (1990) instability analysis of twin jet. • Wlezien (1987) Parameters influencing interactions. • Shaw (1990) Methods to suppress twinjet screech. • Raman, Taghavi (1996, 98) coupling modes, relation to shock cell spacing, etc. • Panickar, Srinivasan, and Raman (2004) Twin jets from two single beveled nozzles. • Joshi, Panickar, Srinivasan, and Raman (2006) Nozzle orientation effects and non-linearity 15
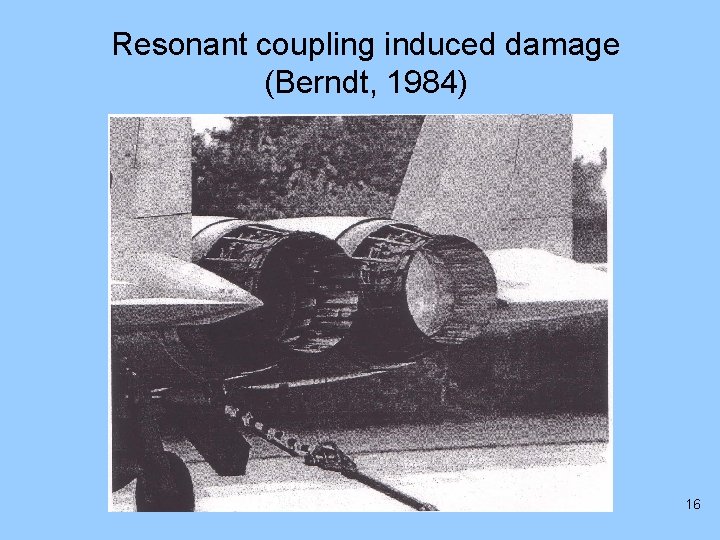
Resonant coupling induced damage (Berndt, 1984) 16
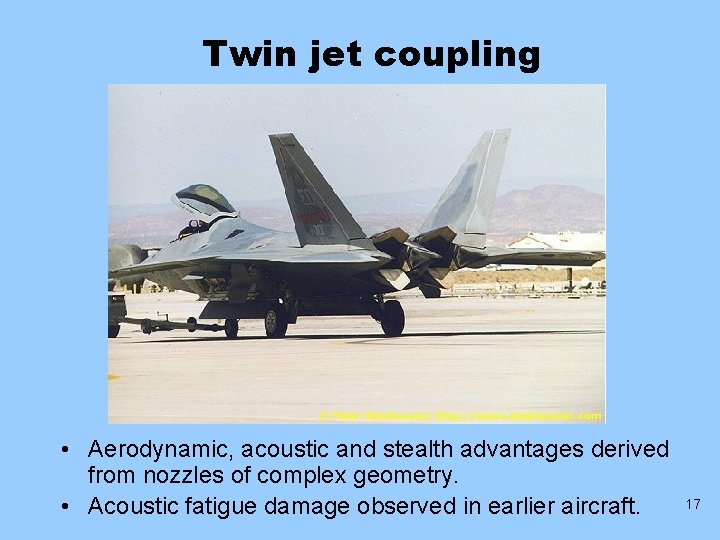
Twin jet coupling • Aerodynamic, acoustic and stealth advantages derived from nozzles of complex geometry. • Acoustic fatigue damage observed in earlier aircraft. 17
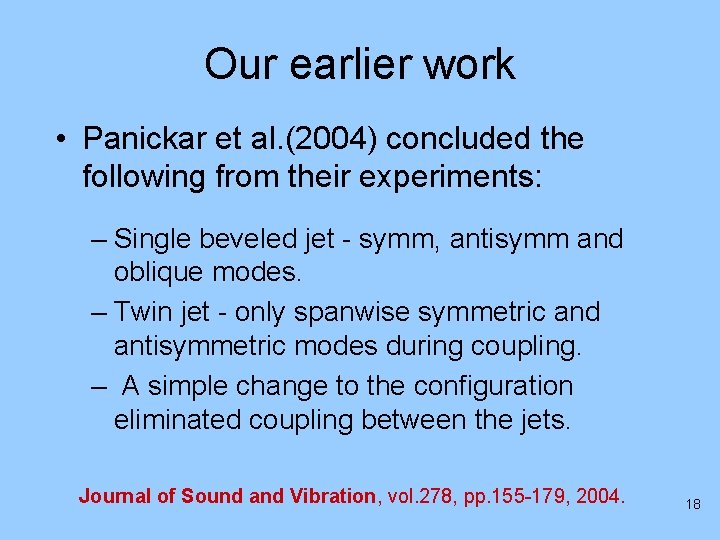
Our earlier work • Panickar et al. (2004) concluded the following from their experiments: – Single beveled jet - symm, antisymm and oblique modes. – Twin jet - only spanwise symmetric and antisymmetric modes during coupling. – A simple change to the configuration eliminated coupling between the jets. Journal of Sound and Vibration, vol. 278, pp. 155 -179, 2004. 18
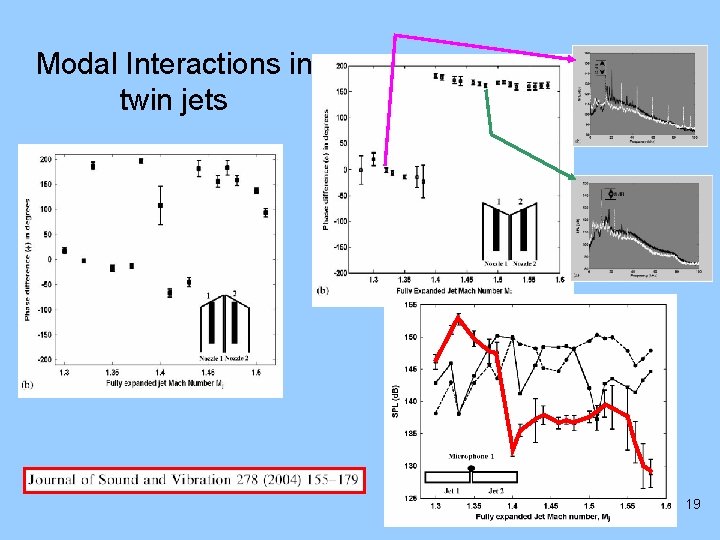
Modal Interactions in twin jets 19
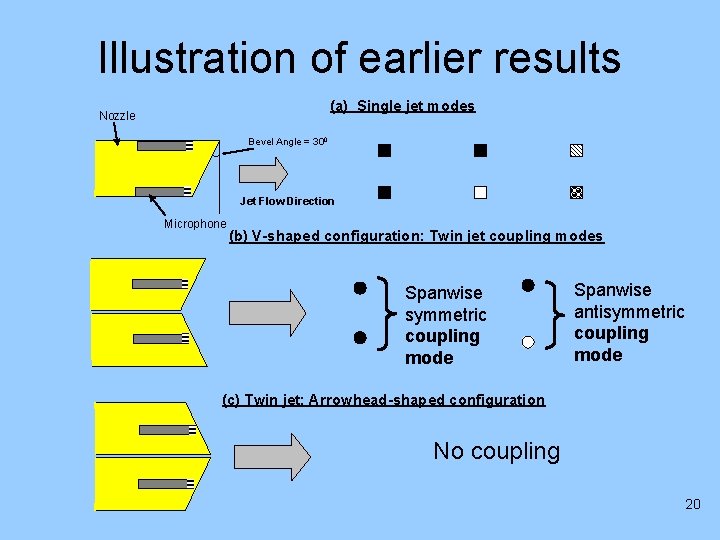
Illustration of earlier results (a) Single jet modes Nozzle Bevel Angle = 300 Jet Flow Direction Microphone (b) V-shaped configuration: Twin jet coupling modes Spanwise symmetric coupling mode Spanwise antisymmetric coupling mode (c) Twin jet: Arrowhead-shaped configuration No coupling 20
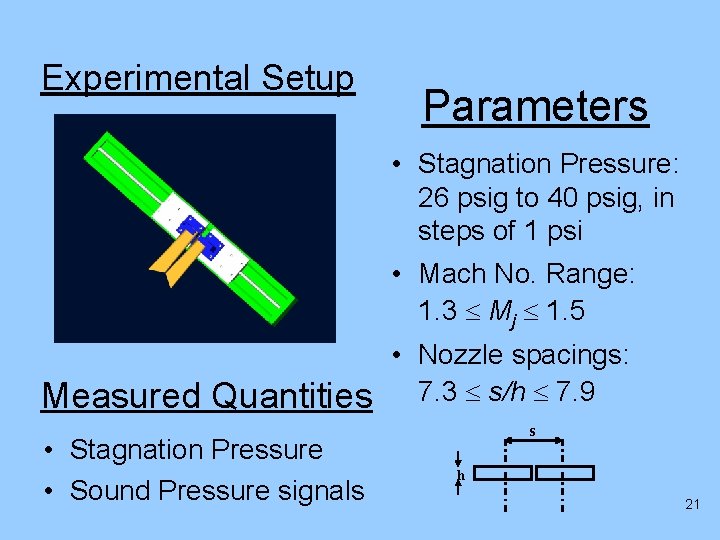
Experimental Setup Parameters • Stagnation Pressure: 26 psig to 40 psig, in steps of 1 psi • Mach No. Range: 1. 3 Mj 1. 5 Measured Quantities • Stagnation Pressure • Sound Pressure signals • Nozzle spacings: 7. 3 s/h 7. 9 s h 21
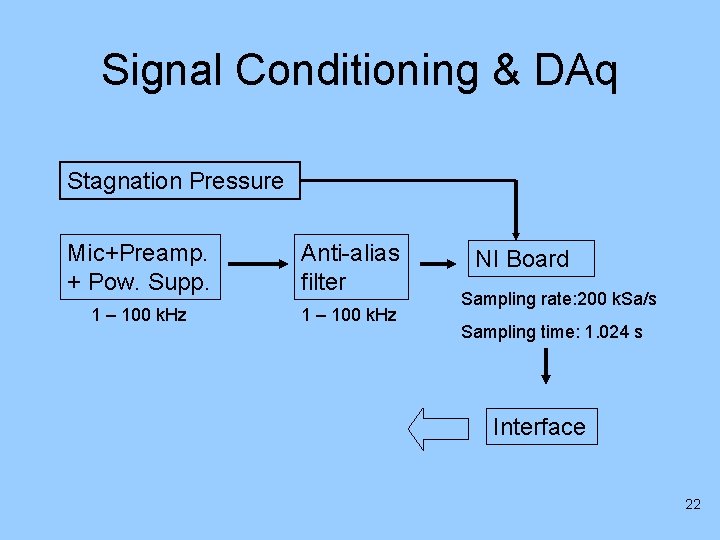
Signal Conditioning & DAq Stagnation Pressure Mic+Preamp. + Pow. Supp. Anti-alias filter 1 – 100 k. Hz NI Board Sampling rate: 200 k. Sa/s Sampling time: 1. 024 s Interface 22
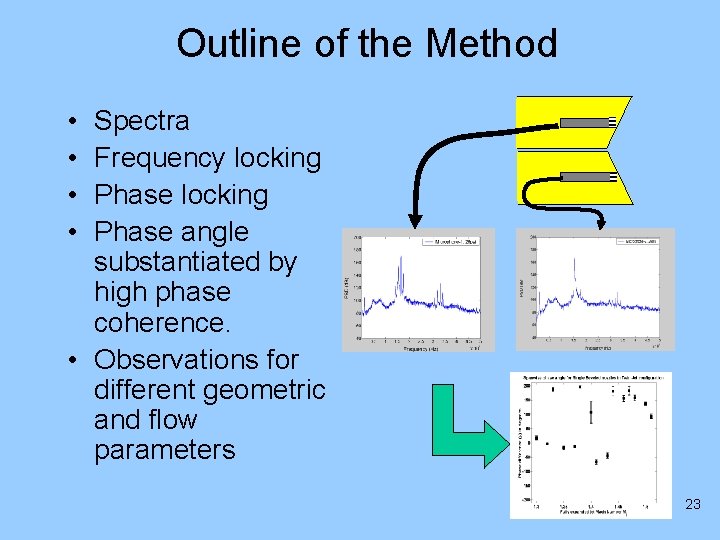
Outline of the Method • • Spectra Frequency locking Phase angle substantiated by high phase coherence. • Observations for different geometric and flow parameters 23
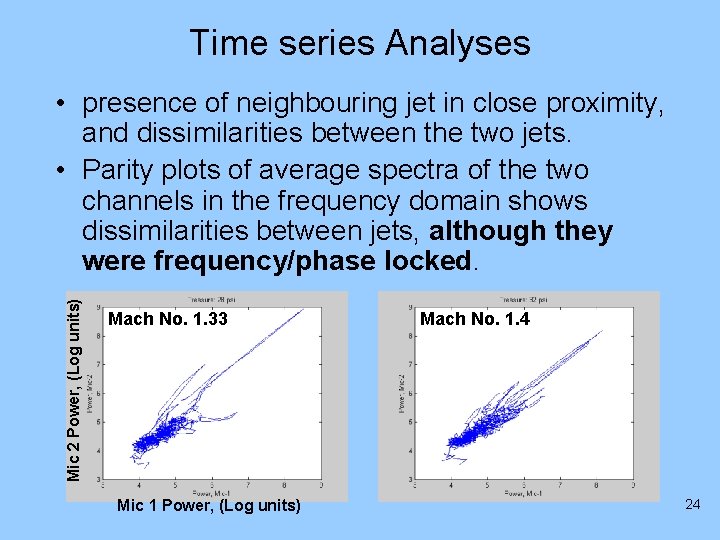
Time series Analyses Mic 2 Power, (Log units) • presence of neighbouring jet in close proximity, and dissimilarities between the two jets. • Parity plots of average spectra of the two channels in the frequency domain shows dissimilarities between jets, although they were frequency/phase locked. Mach No. 1. 33 Mic 1 Power, (Log units) Mach No. 1. 4 24
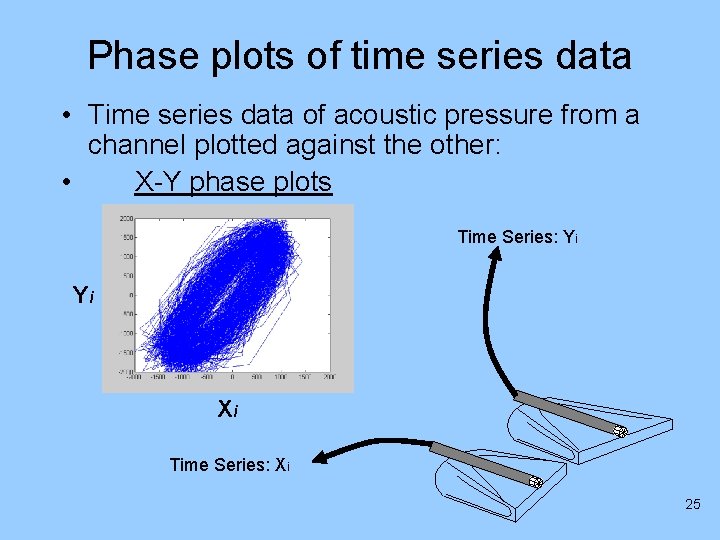
Phase plots of time series data • Time series data of acoustic pressure from a channel plotted against the other: • X-Y phase plots Time Series: Yi Yi Xi Time Series: Xi 25
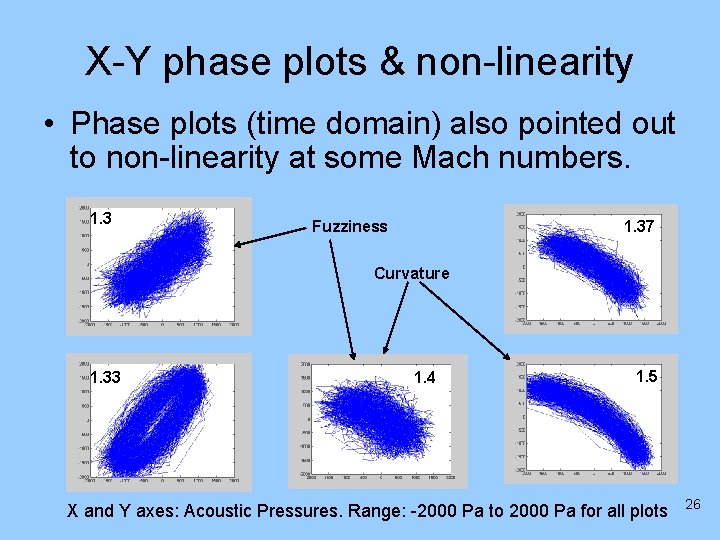
X-Y phase plots & non-linearity • Phase plots (time domain) also pointed out to non-linearity at some Mach numbers. 1. 3 Fuzziness 1. 37 Curvature 1. 33 1. 4 1. 5 X and Y axes: Acoustic Pressures. Range: -2000 Pa to 2000 Pa for all plots 26
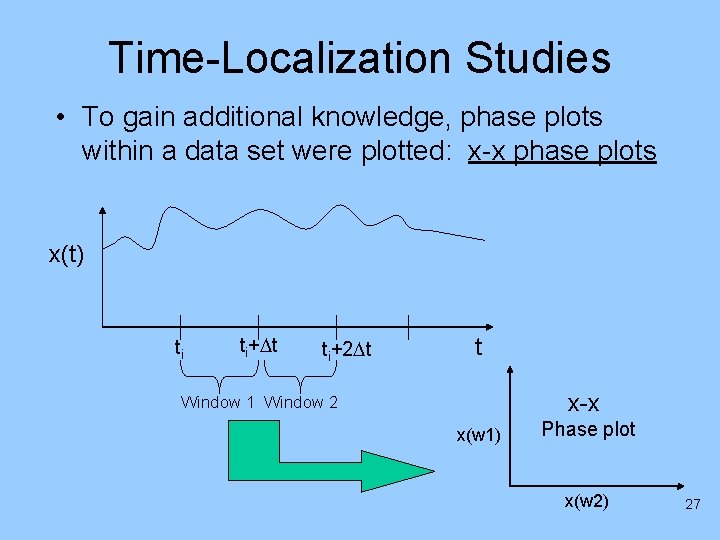
Time-Localization Studies • To gain additional knowledge, phase plots within a data set were plotted: x-x phase plots x(t) ti ti+ t ti+2 t t x-x Window 1 Window 2 x(w 1) Phase plot x(w 2) 27
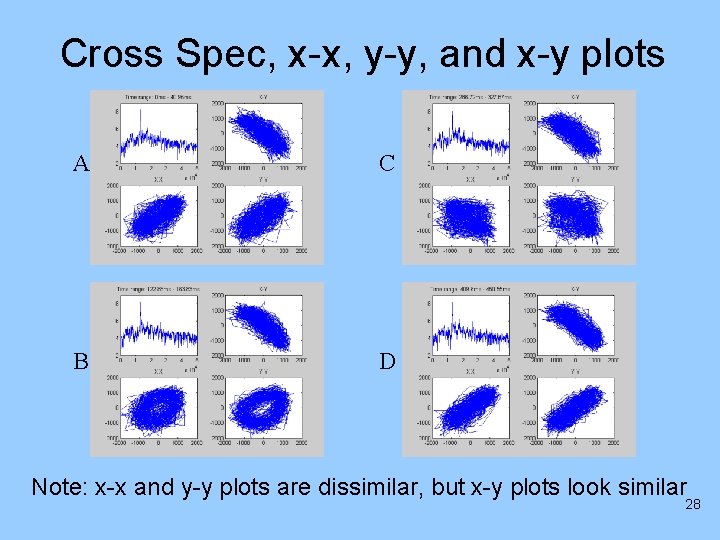
Cross Spec, x-x, y-y, and x-y plots A C B D Note: x-x and y-y plots are dissimilar, but x-y plots look similar 28
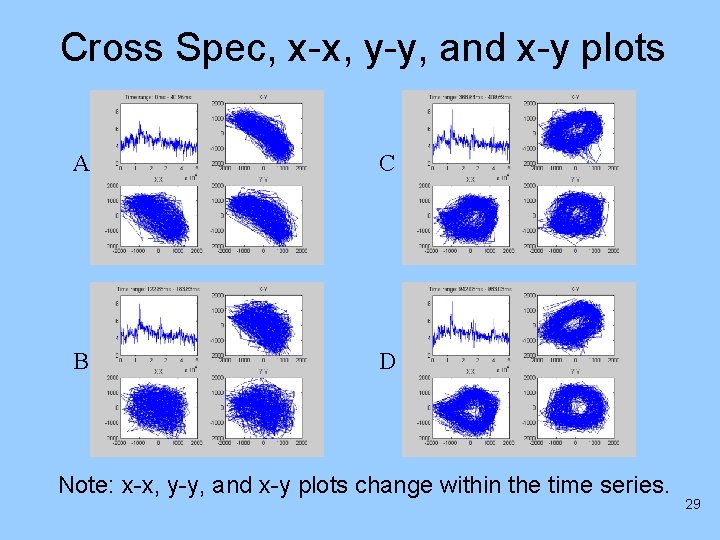
Cross Spec, x-x, y-y, and x-y plots A C B D Note: x-x, y-y, and x-y plots change within the time series. 29
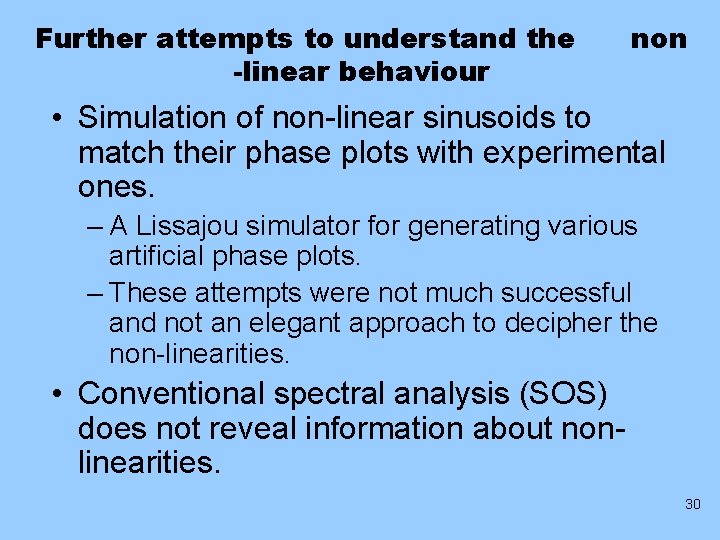
Further attempts to understand the -linear behaviour non • Simulation of non-linear sinusoids to match their phase plots with experimental ones. – A Lissajou simulator for generating various artificial phase plots. – These attempts were not much successful and not an elegant approach to decipher the non-linearities. • Conventional spectral analysis (SOS) does not reveal information about nonlinearities. 30
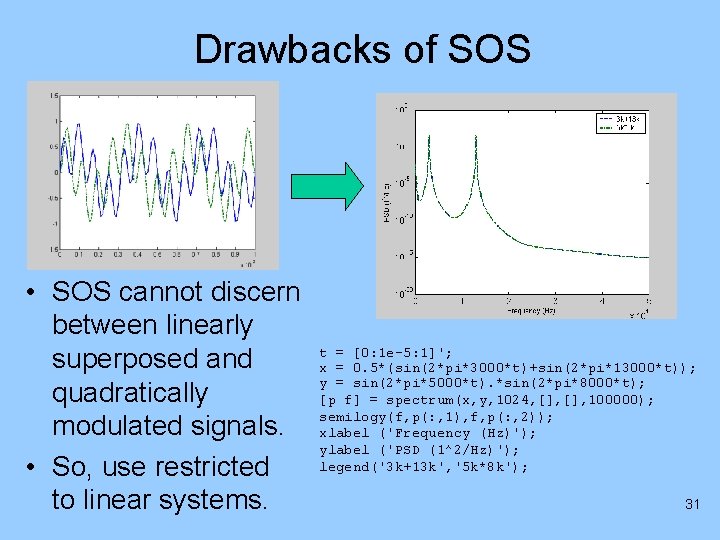
Drawbacks of SOS • SOS cannot discern between linearly superposed and quadratically modulated signals. • So, use restricted to linear systems. t = [0: 1 e-5: 1]'; x = 0. 5*(sin(2*pi*3000*t)+sin(2*pi*13000*t)); y = sin(2*pi*5000*t). *sin(2*pi*8000*t); [p f] = spectrum(x, y, 1024, [], 100000); semilogy(f, p(: , 1), f, p(: , 2)); xlabel ('Frequency (Hz)'); ylabel ('PSD (1^2/Hz)'); legend('3 k+13 k', '5 k*8 k'); 31
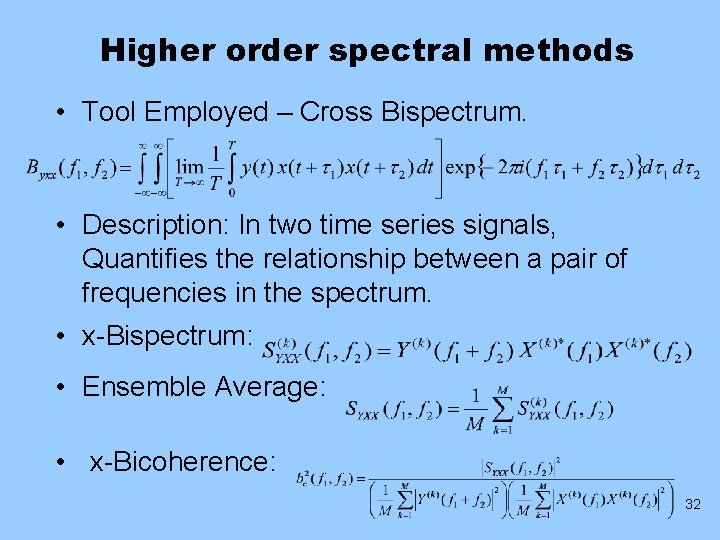
Higher order spectral methods • Tool Employed – Cross Bispectrum. • Description: In two time series signals, Quantifies the relationship between a pair of frequencies in the spectrum. • x-Bispectrum: • Ensemble Average: • x-Bicoherence: 32
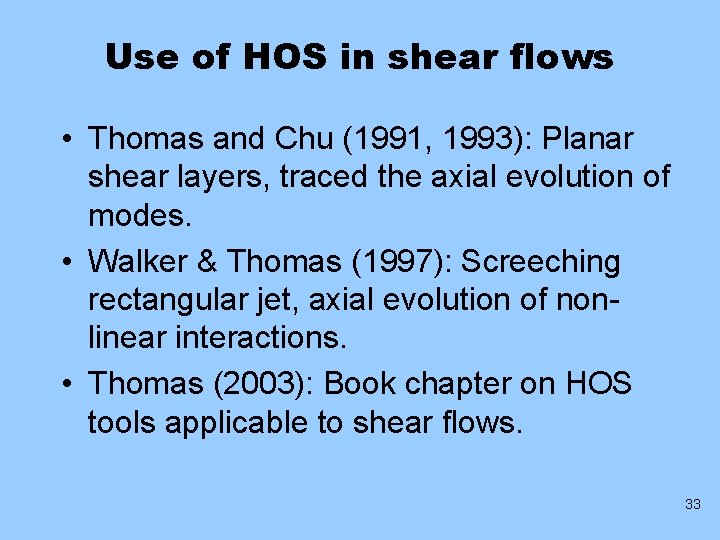
Use of HOS in shear flows • Thomas and Chu (1991, 1993): Planar shear layers, traced the axial evolution of modes. • Walker & Thomas (1997): Screeching rectangular jet, axial evolution of nonlinear interactions. • Thomas (2003): Book chapter on HOS tools applicable to shear flows. 33
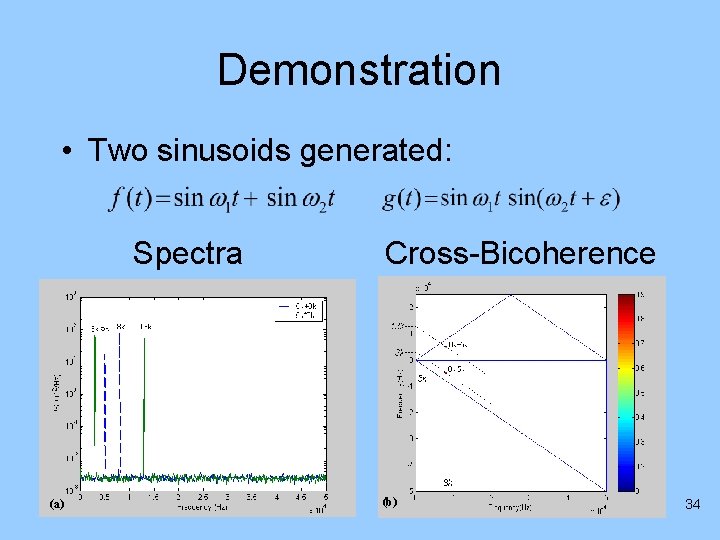
Demonstration • Two sinusoids generated: Spectra (a) Cross-Bicoherence (b) 34
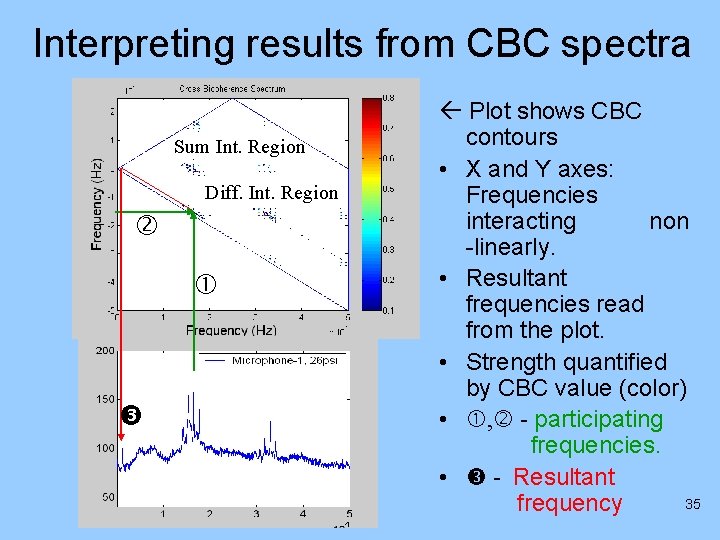
Interpreting results from CBC spectra Sum Int. Region Diff. Int. Region Plot shows CBC contours • X and Y axes: Frequencies interacting non -linearly. • Resultant frequencies read from the plot. • Strength quantified by CBC value (color) • , - participating frequencies. • - Resultant 35 frequency
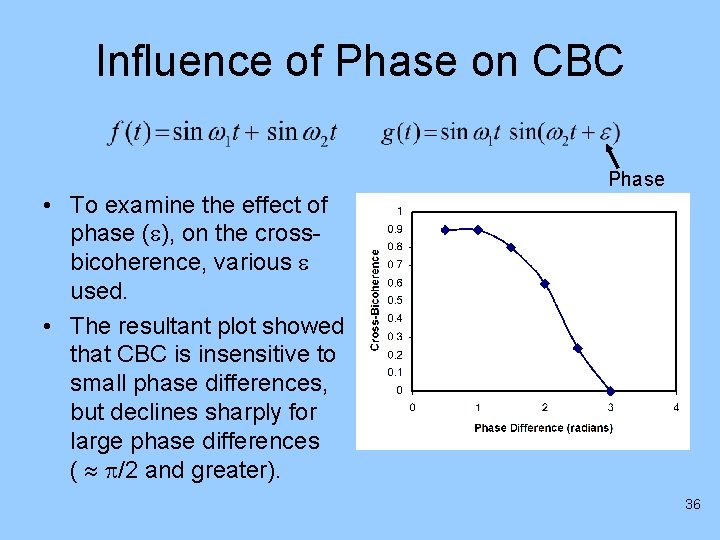
Influence of Phase on CBC Phase • To examine the effect of phase ( ), on the crossbicoherence, various used. • The resultant plot showed that CBC is insensitive to small phase differences, but declines sharply for large phase differences ( /2 and greater). 36
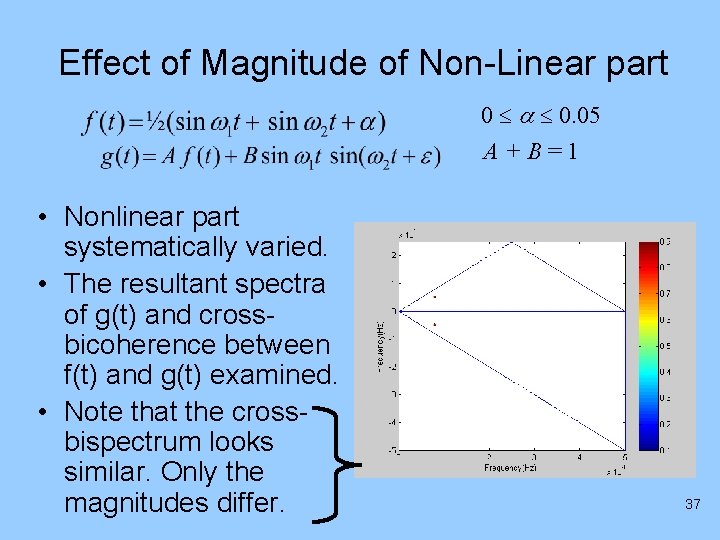
Effect of Magnitude of Non-Linear part 0 0. 05 A + B = 1 • Nonlinear part systematically varied. • The resultant spectra of g(t) and crossbicoherence between f(t) and g(t) examined. • Note that the crossbispectrum looks similar. Only the magnitudes differ. 37
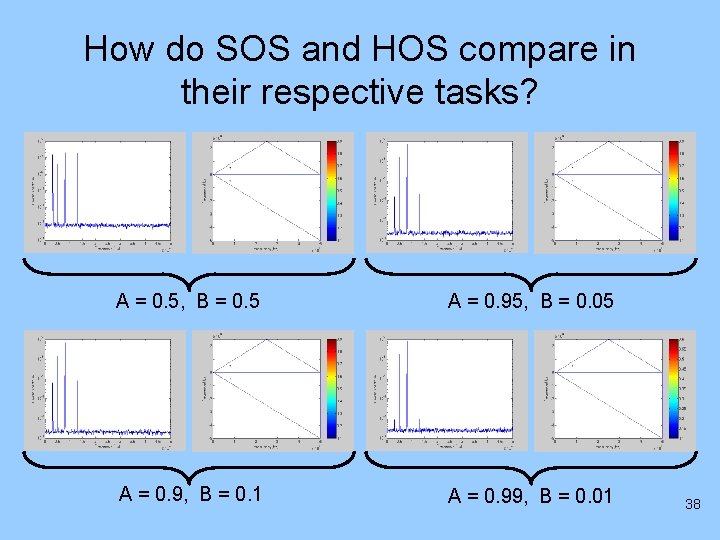
How do SOS and HOS compare in their respective tasks? A = 0. 5, B = 0. 5 A = 0. 95, B = 0. 05 A = 0. 9, B = 0. 1 A = 0. 99, B = 0. 01 38
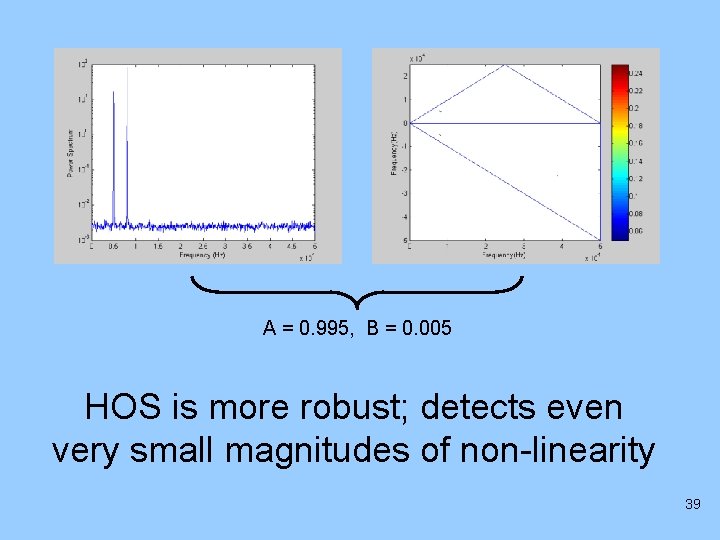
A = 0. 995, B = 0. 005 HOS is more robust; detects even very small magnitudes of non-linearity 39
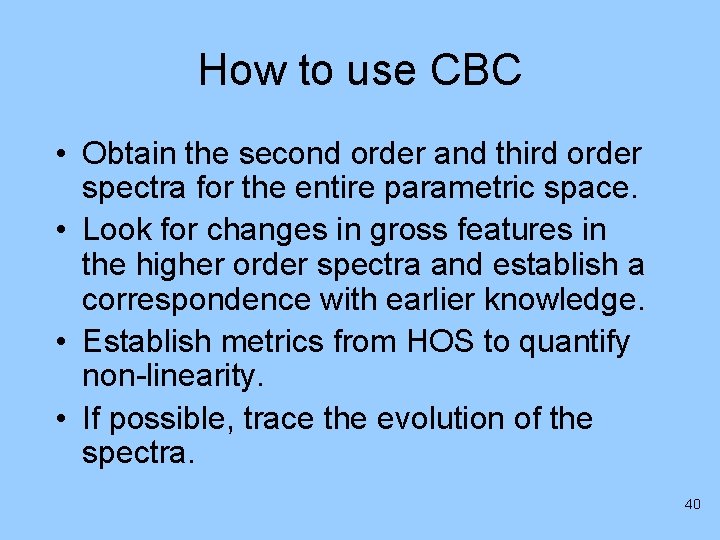
How to use CBC • Obtain the second order and third order spectra for the entire parametric space. • Look for changes in gross features in the higher order spectra and establish a correspondence with earlier knowledge. • Establish metrics from HOS to quantify non-linearity. • If possible, trace the evolution of the spectra. 40
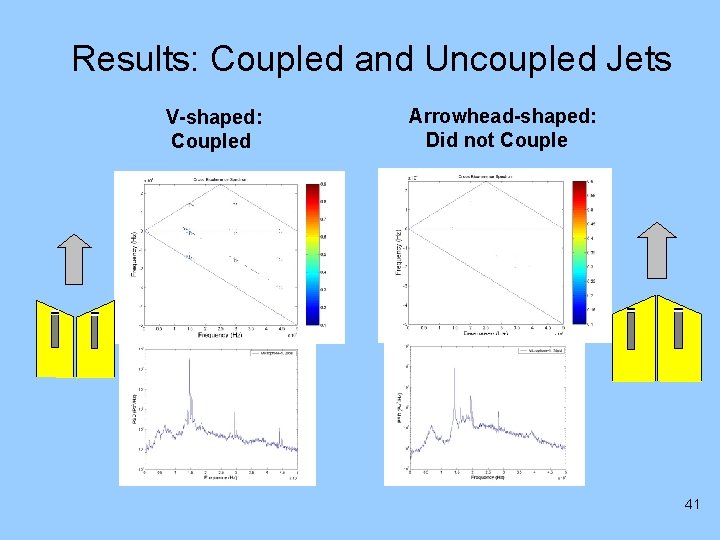
Results: Coupled and Uncoupled Jets Arrowhead-shaped: Did not Couple V-shaped: Coupled 41
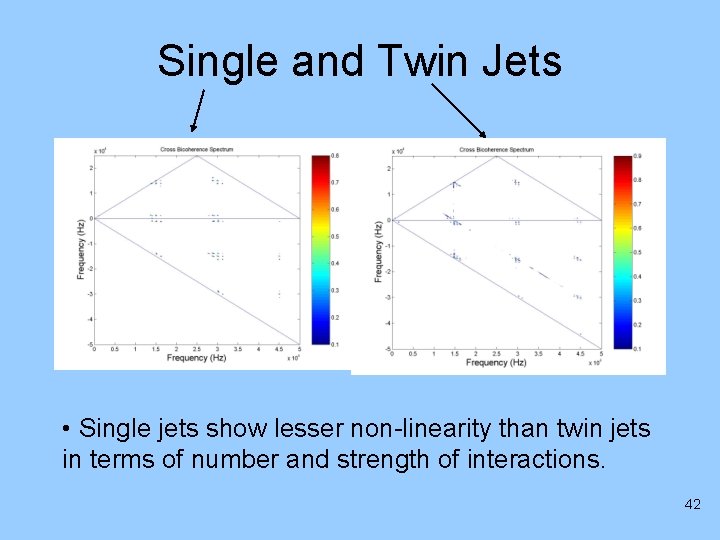
Single and Twin Jets • Single jets show lesser non-linearity than twin jets in terms of number and strength of interactions. 42
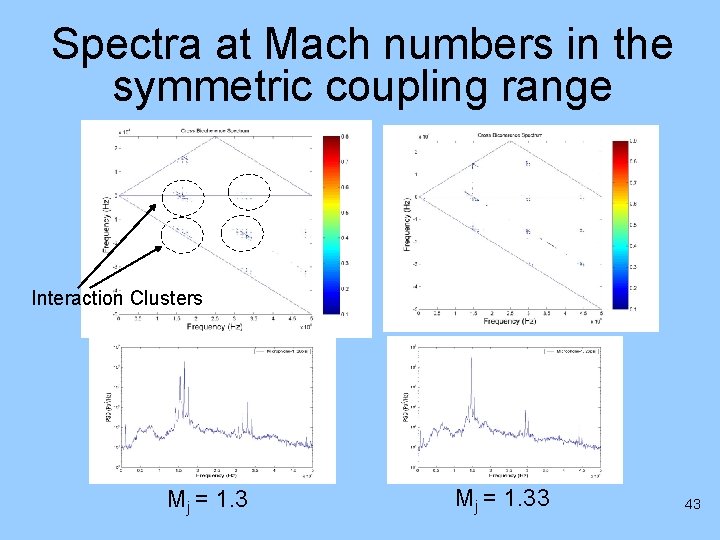
Spectra at Mach numbers in the symmetric coupling range Interaction Clusters Mj = 1. 33 43
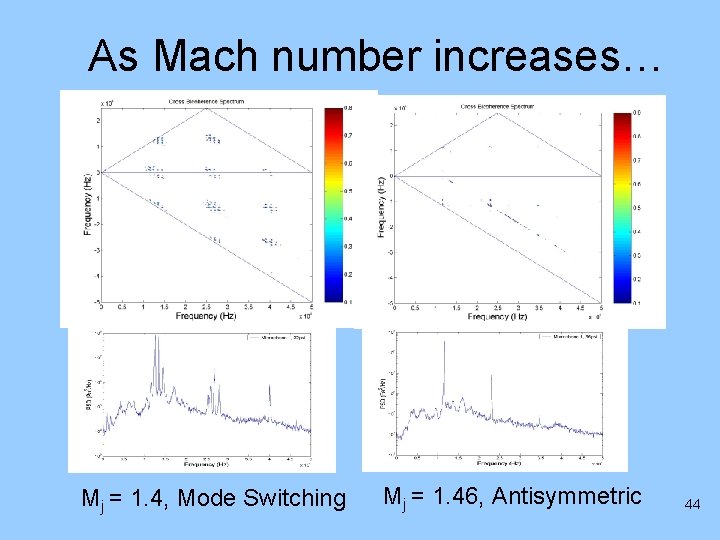
As Mach number increases… Mj = 1. 4, Mode Switching Mj = 1. 46, Antisymmetric 44
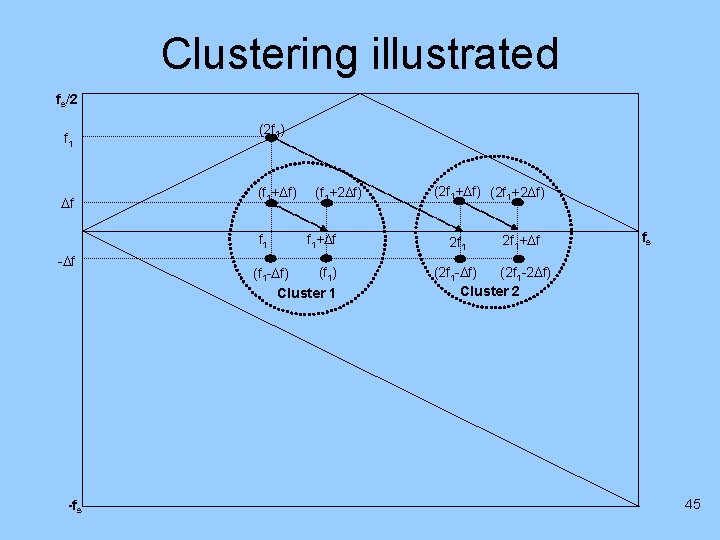
Clustering illustrated fs/2 f 1 f (2 f 1) (f 1+ f) f 1 - f -fs (f 1+2 f) f 1+ f (f 1) (f 1 - f) Cluster 1 (2 f 1+ f) (2 f 1+2 f) 2 f 1+ f fs (2 f 1 -2 f) (2 f 1 - f) Cluster 2 45
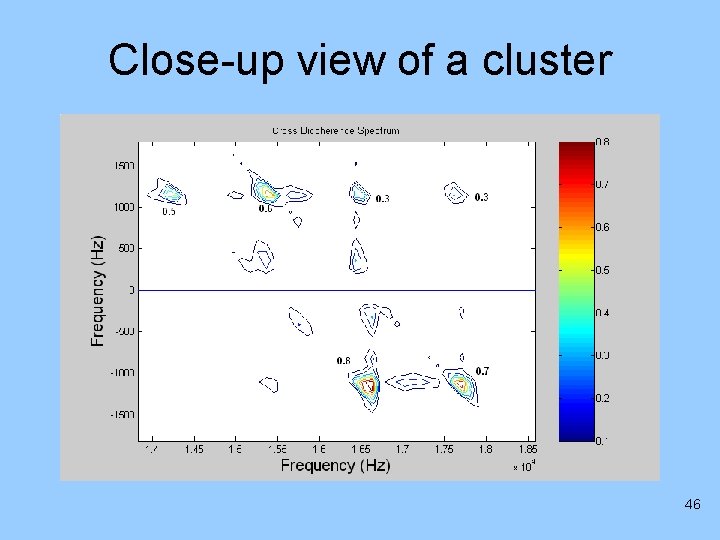
Close-up view of a cluster 46
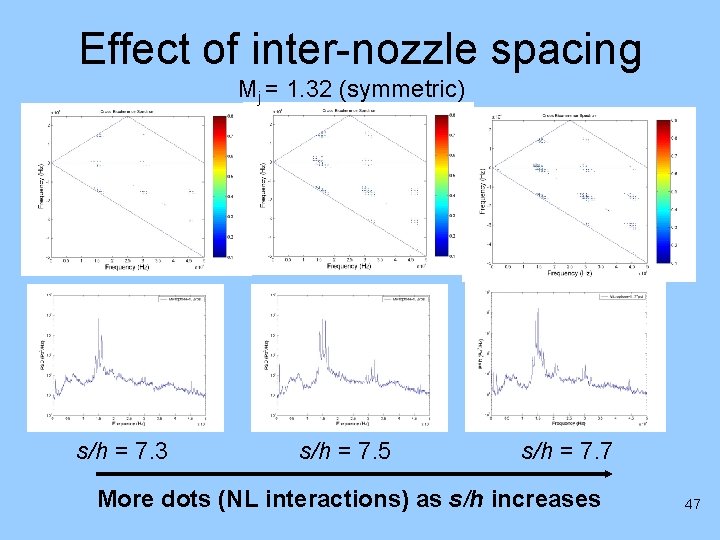
Effect of inter-nozzle spacing Mj = 1. 32 (symmetric) s/h = 7. 3 s/h = 7. 5 s/h = 7. 7 More dots (NL interactions) as s/h increases 47
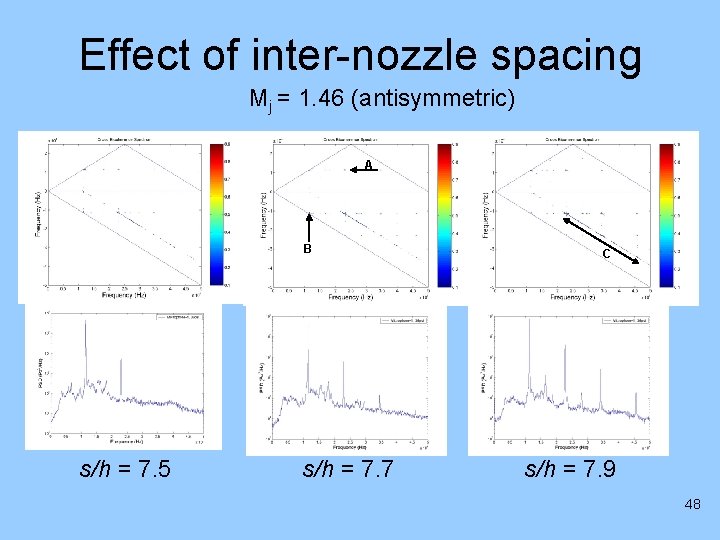
Effect of inter-nozzle spacing Mj = 1. 46 (antisymmetric) A B s/h = 7. 5 s/h = 7. 7 C s/h = 7. 9 48
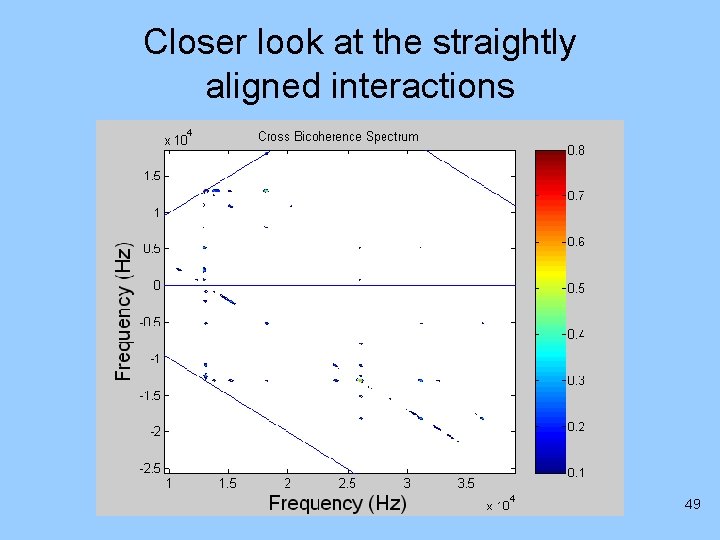
Closer look at the straightly aligned interactions 49
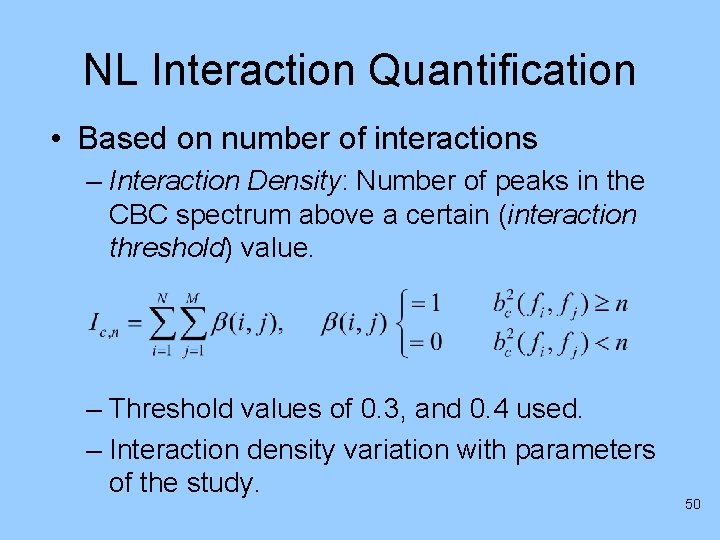
NL Interaction Quantification • Based on number of interactions – Interaction Density: Number of peaks in the CBC spectrum above a certain (interaction threshold) value. – Threshold values of 0. 3, and 0. 4 used. – Interaction density variation with parameters of the study. 50
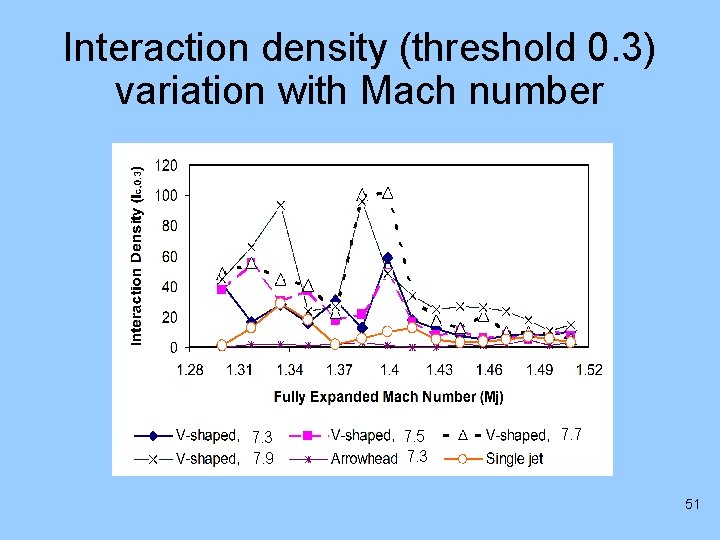
Interaction density (threshold 0. 3) variation with Mach number 7. 3 7. 9 7. 5 7. 3 7. 7 51
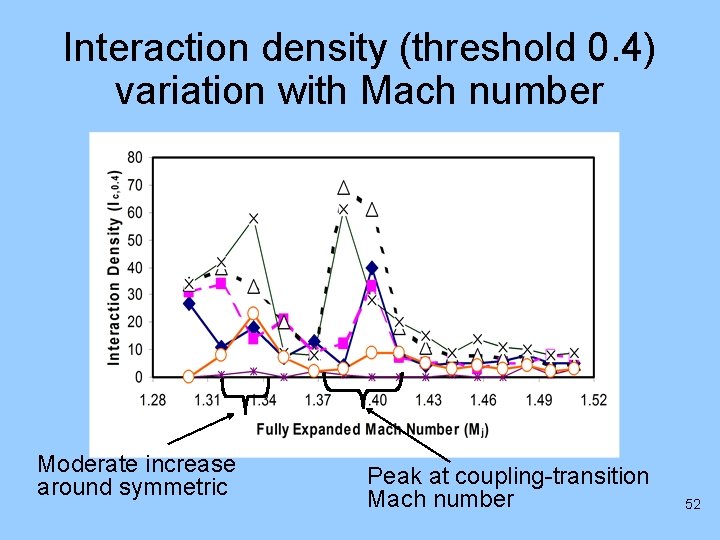
Interaction density (threshold 0. 4) variation with Mach number Moderate increase around symmetric Peak at coupling-transition Mach number 52
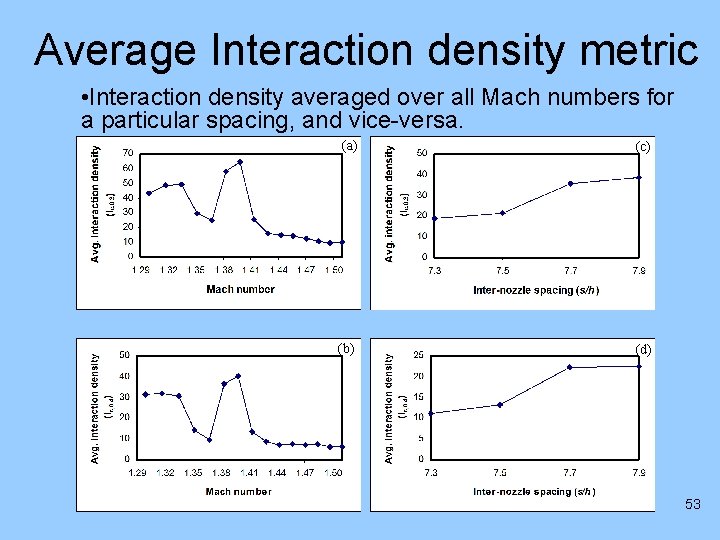
Average Interaction density metric • Interaction density averaged over all Mach numbers for a particular spacing, and vice-versa. (a) (c) (b) (d) 53
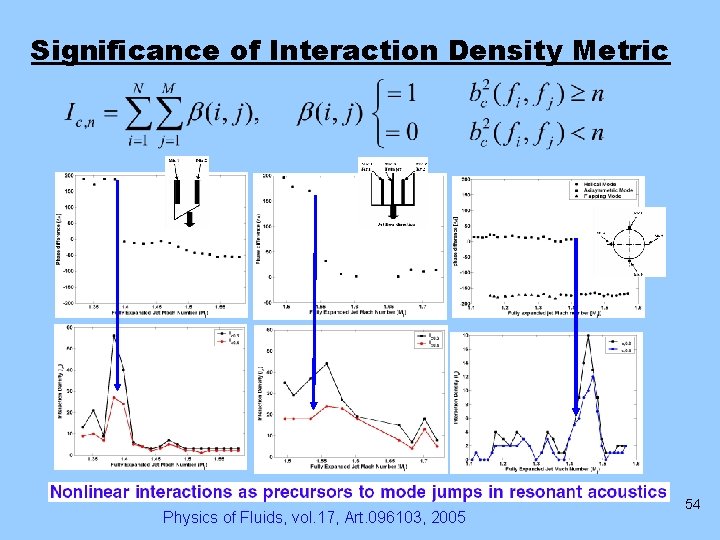
Significance of Interaction Density Metric Physics of Fluids, vol. 17, Art. 096103, 2005 54
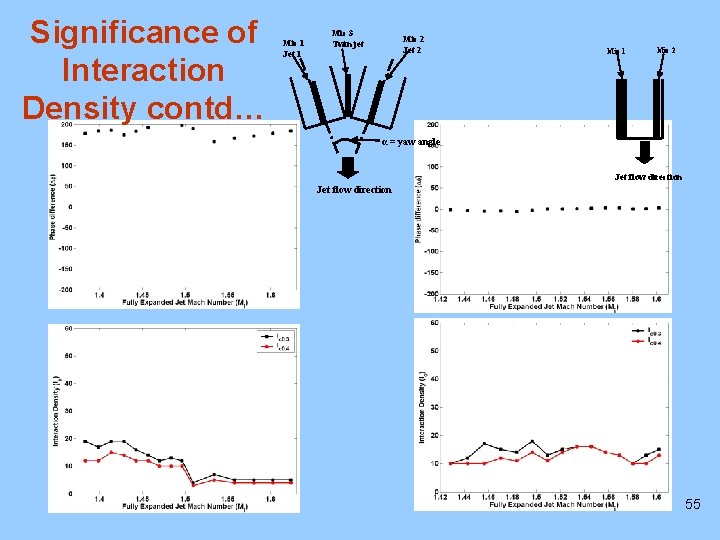
Significance of Interaction Density contd… Mic 1 Jet 1 Mic 3 Twin jet Mic 2 Jet 2 Mic 1 Mic 2 α = yaw angle Jet flow direction 55
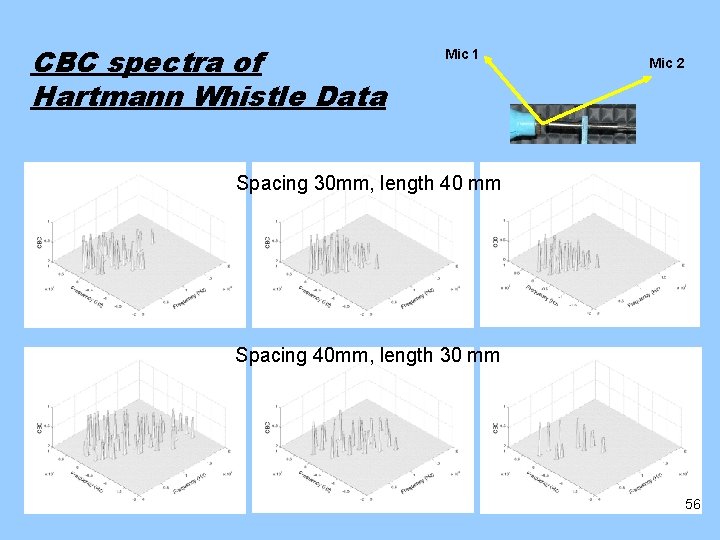
CBC spectra of Hartmann Whistle Data Mic 1 Mic 2 Spacing 30 mm, length 40 mm Spacing 40 mm, length 30 mm 56
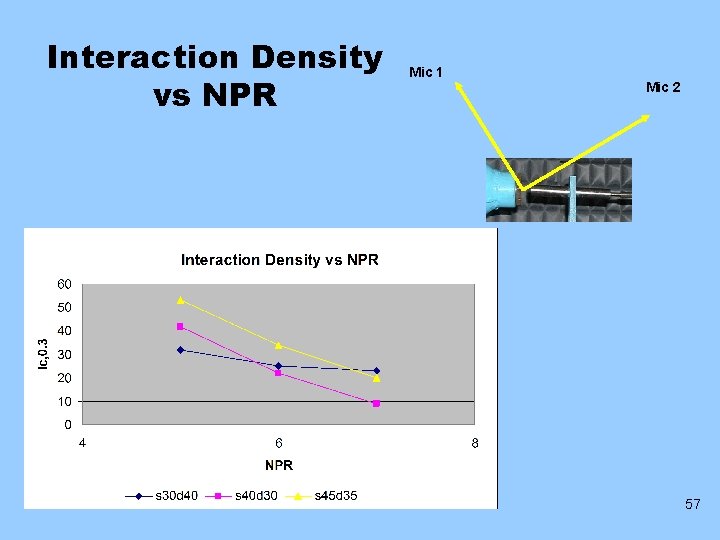
Interaction Density vs NPR Mic 1 Mic 2 57
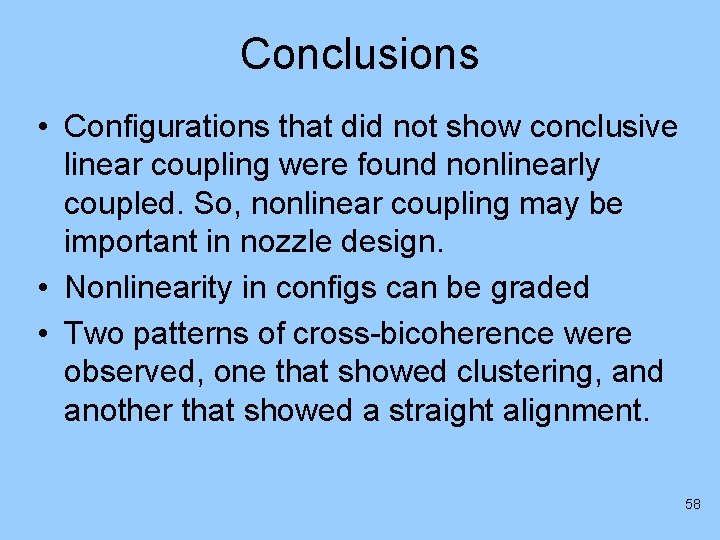
Conclusions • Configurations that did not show conclusive linear coupling were found nonlinearly coupled. So, nonlinear coupling may be important in nozzle design. • Nonlinearity in configs can be graded • Two patterns of cross-bicoherence were observed, one that showed clustering, and another that showed a straight alignment. 58
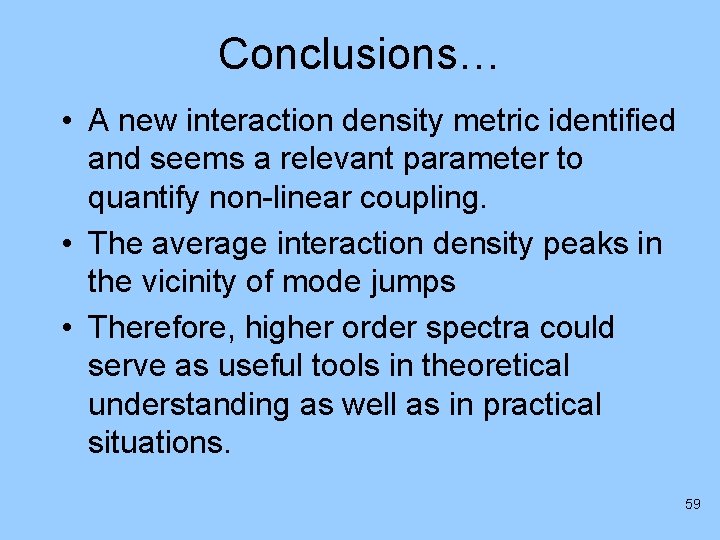
Conclusions… • A new interaction density metric identified and seems a relevant parameter to quantify non-linear coupling. • The average interaction density peaks in the vicinity of mode jumps • Therefore, higher order spectra could serve as useful tools in theoretical understanding as well as in practical situations. 59
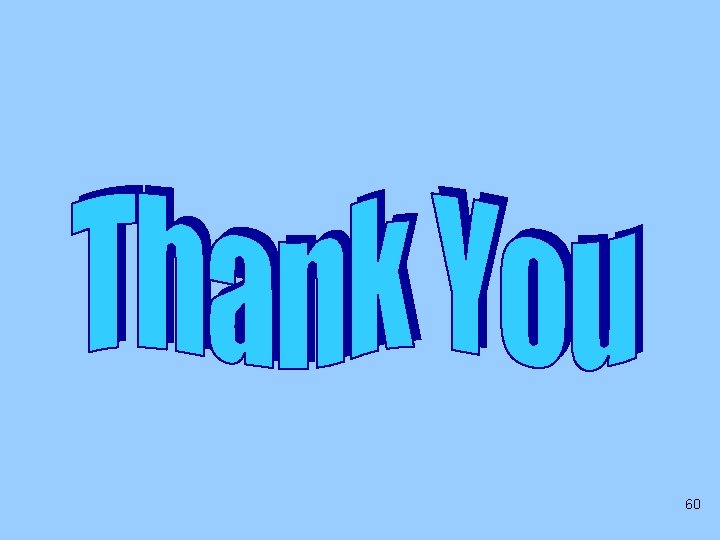
60
Vernier spectroscopy
Multitaper spectral analysis
C srinivasan waste management
Madhumitha srinivasan
N srinivasan mbu iisc
Nanda srinivasan
Siva srinivasan
Dr k srinivasan
Sharadha srinivasan
Srinivasan seshadri
Sathish srinivasan
Jayanthi srinivasan
Srinivasan seshan
Introduction to nonlinear analysis
Bilinear isotropic hardening ansys
Differences between linear and nonlinear equations
Spectral regrowth
Spectral regrowth
Spectral classification
Profil spectral de rigel
Spectral normalization
Hashing
Spectral graph theory applications
Spectral efficiency
Domaine spectral
Spectral leakage
Spectral bands
Eric xing
Spectral clustering
Video spectral comparator (vsc)
Spectral clustering
Spectral characteristics of angle modulated signals
Analytical spectral devices
Theorem
Value at risk formula
Spectral graph theory and its applications
Non rigid rotator
Rotational spectral lines
Rotational spectral lines
Spectral karyotype
Meridional rays in optical fiber
Adobe audition pitch shifter
Spectral graph
Absolute magnitude to luminosity
Neon spectral lines
Potassium lithium
Spectral graph theory spielman
Sid and oid
Spectral class
Which is one physical property that all stars have?
Spectral sensitivity
Spectral leakage
Global spectral model
Psd of ask
Spectral classes
Minimum distance classifier
Spectral transformation of iir filters
Spectral imaging
Spectral unmixing
Atomic spectral lines
Matting levin