Chapter 16 Respiratory System Part 2 Copyright The
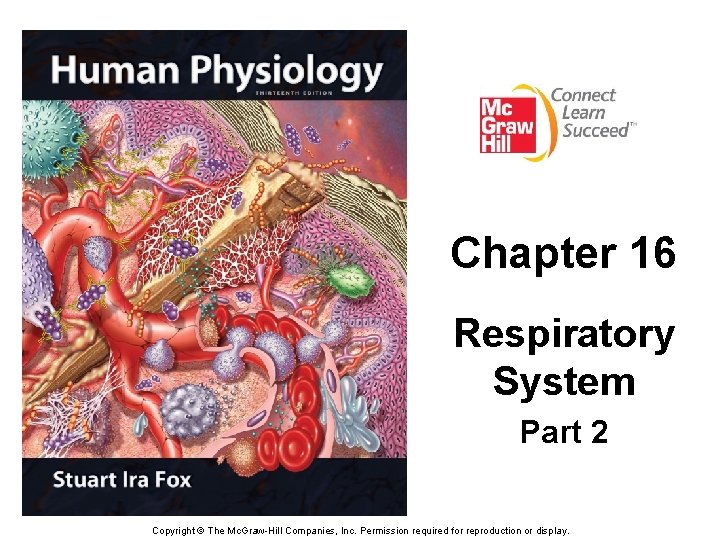
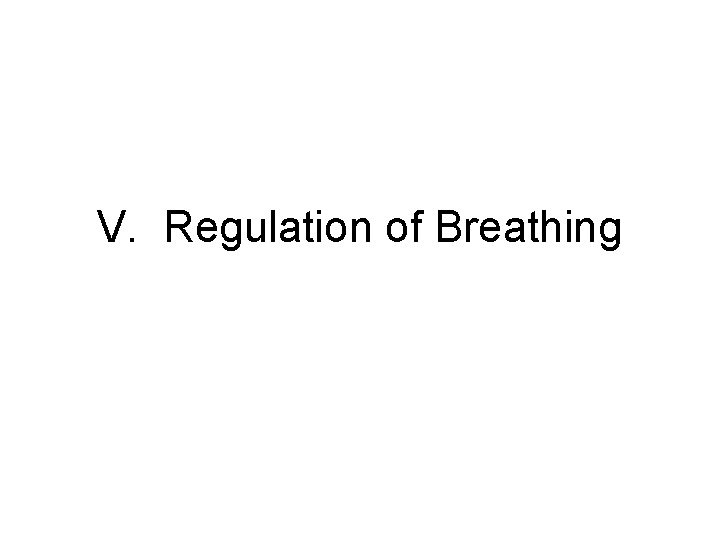
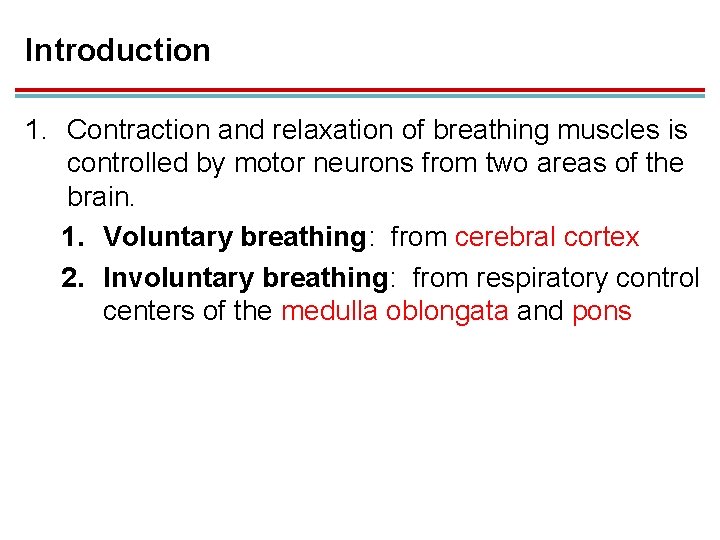
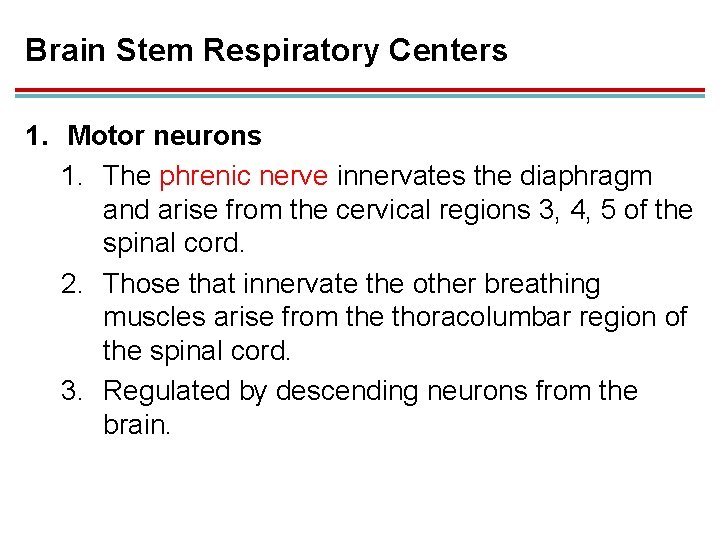
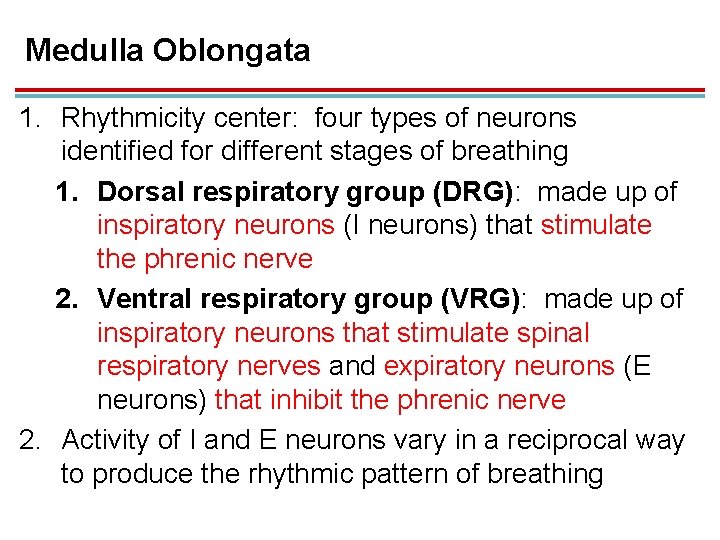
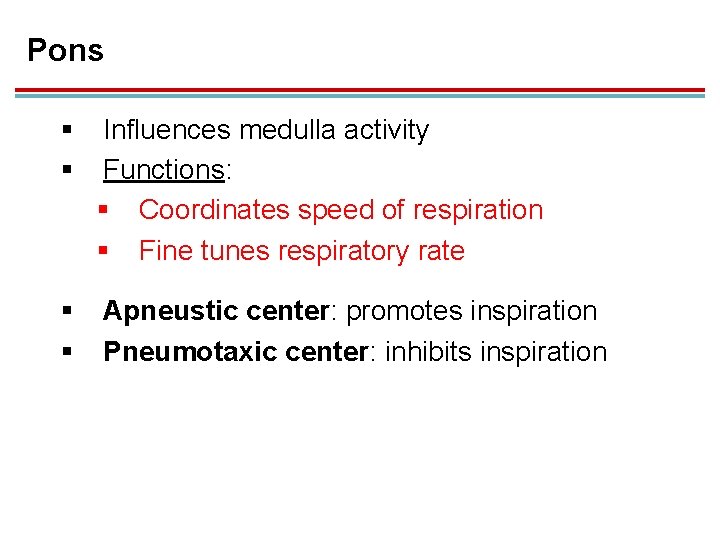
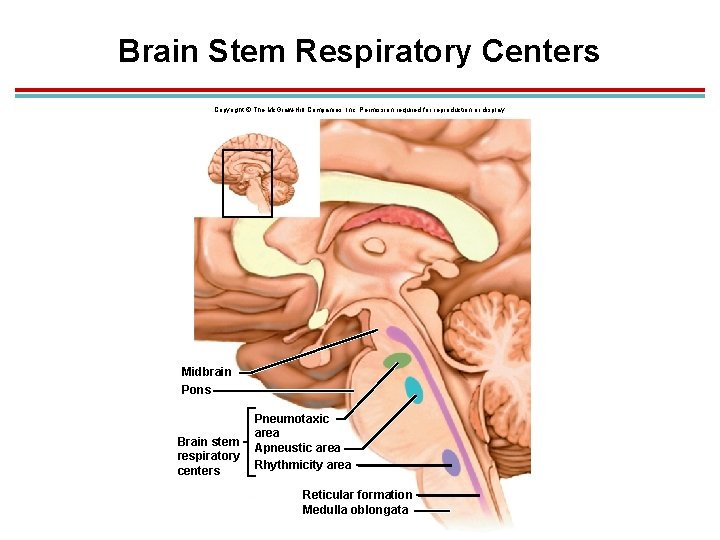
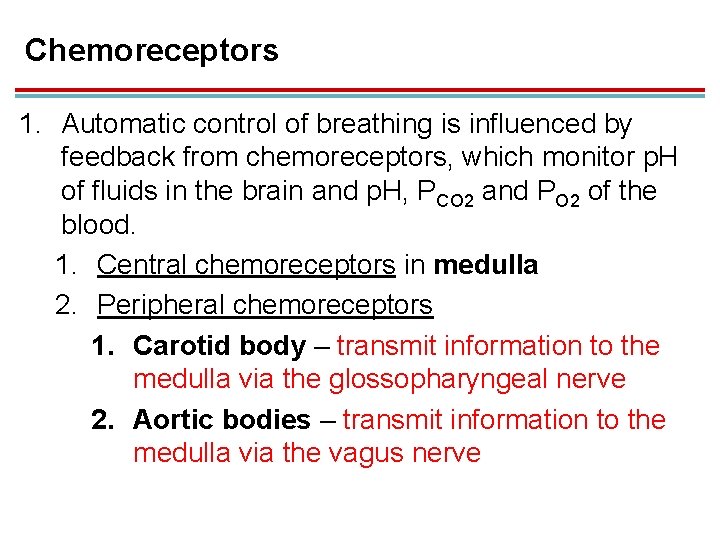
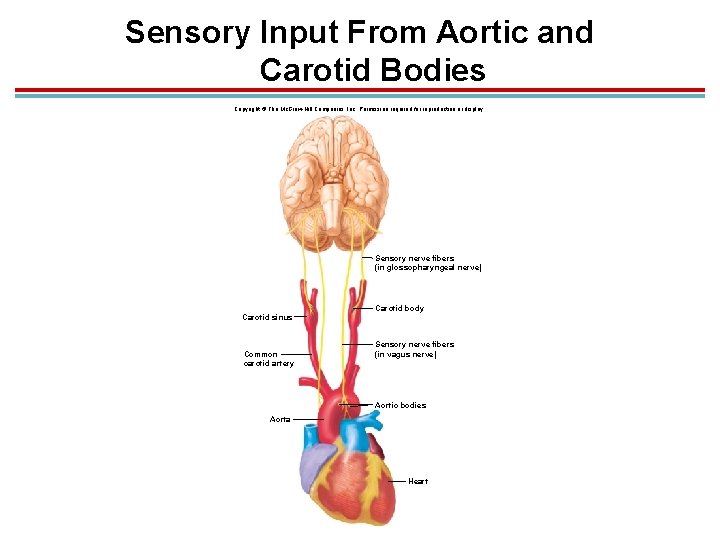
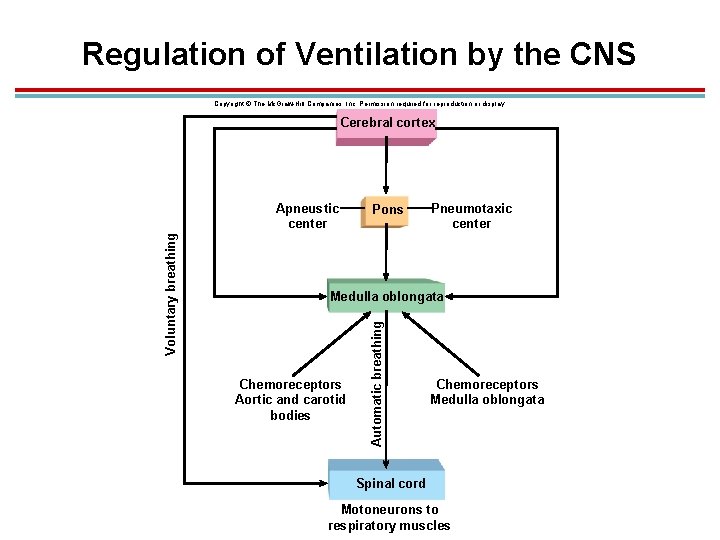
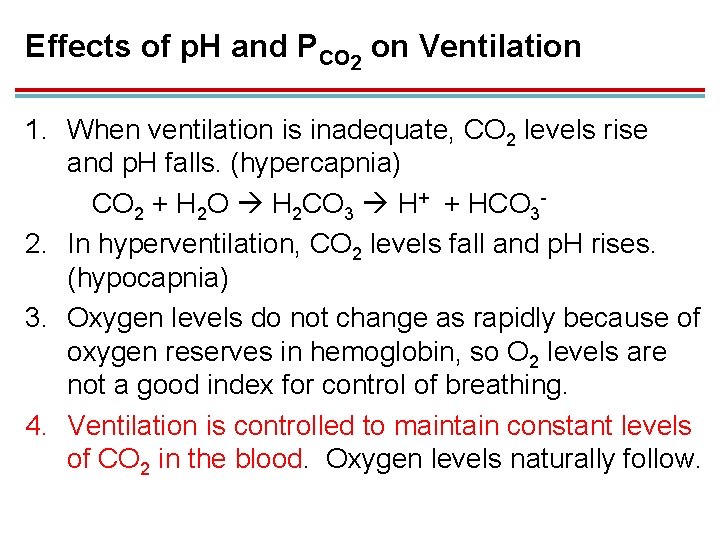
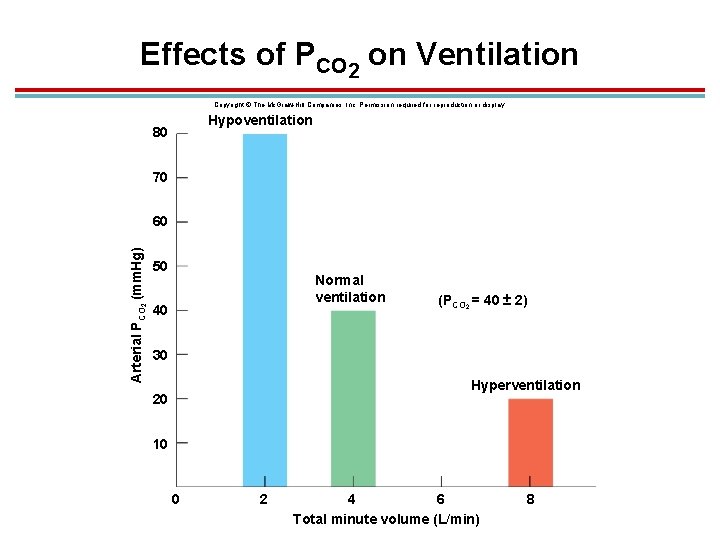
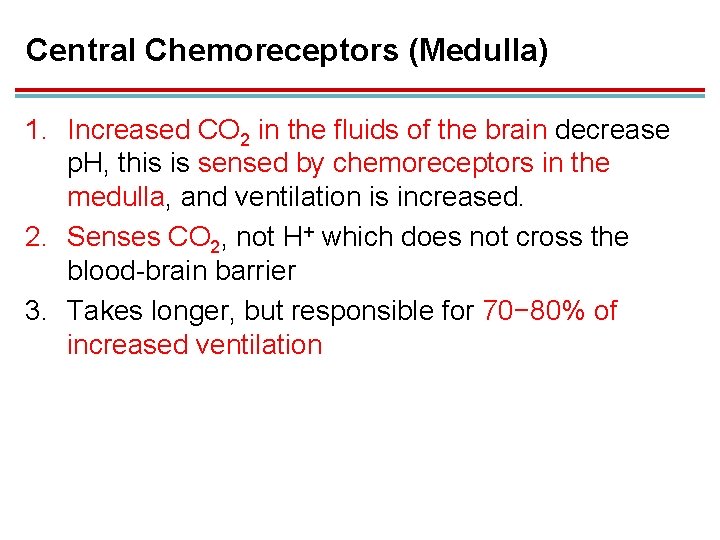
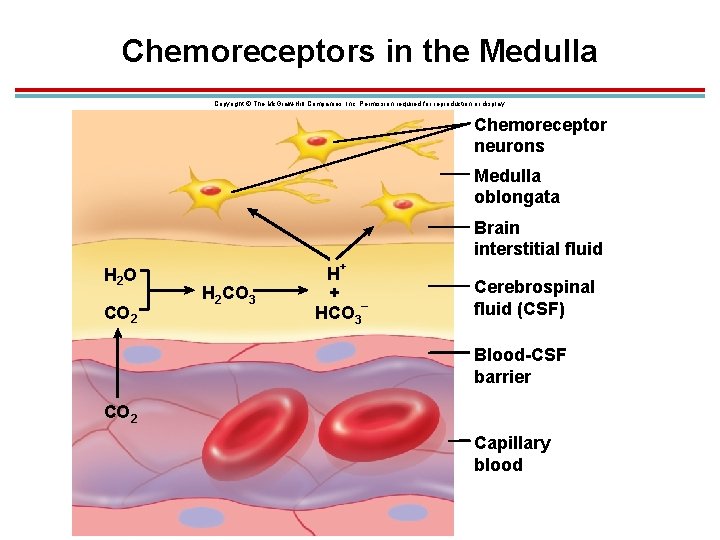
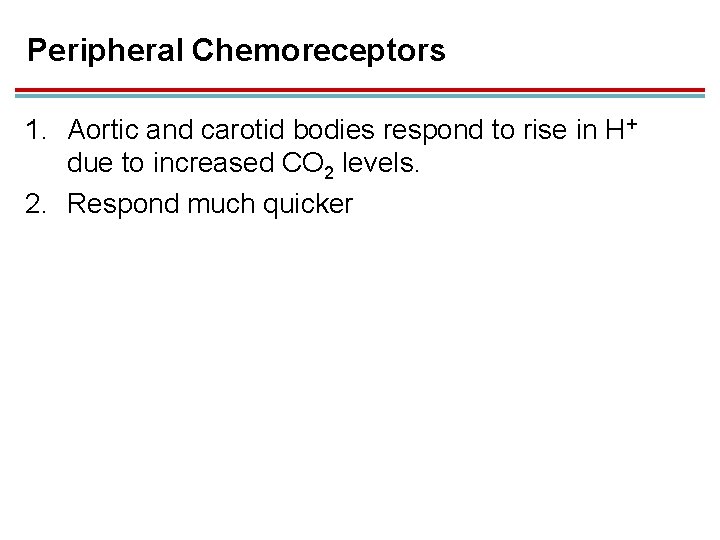
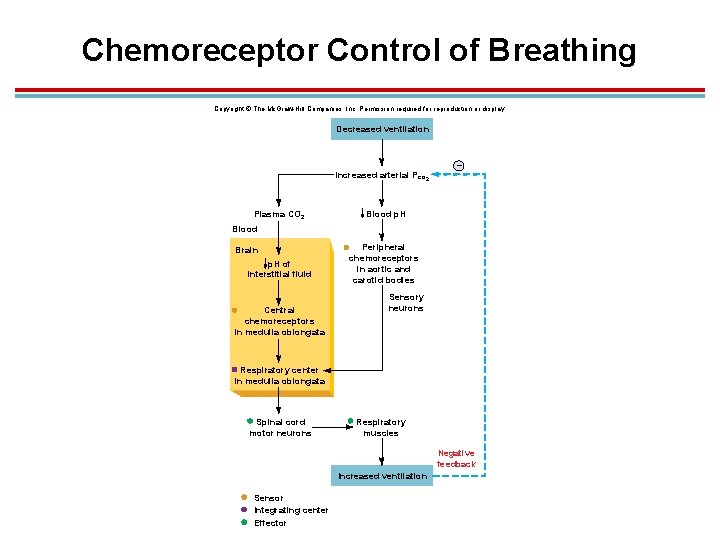
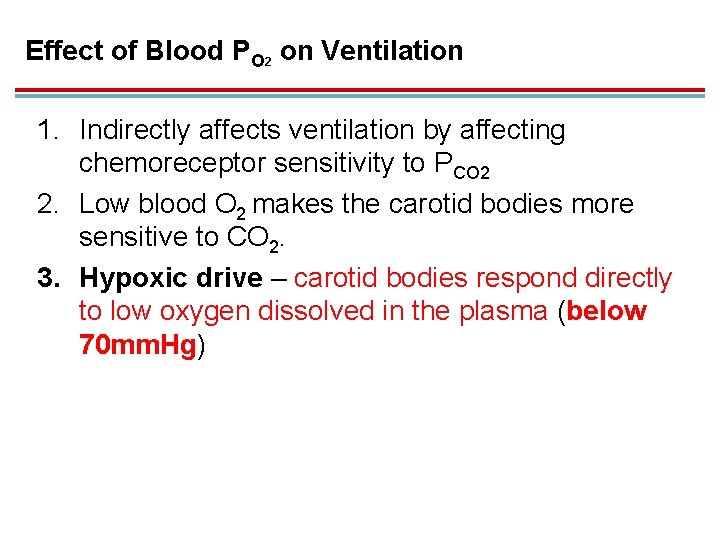
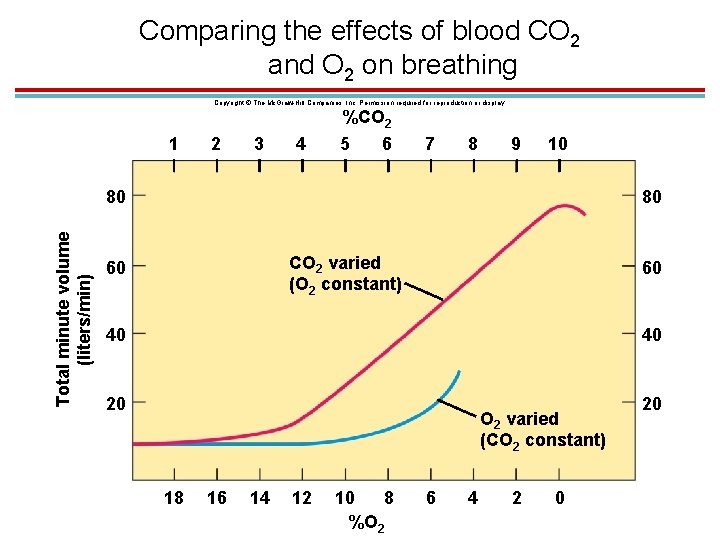
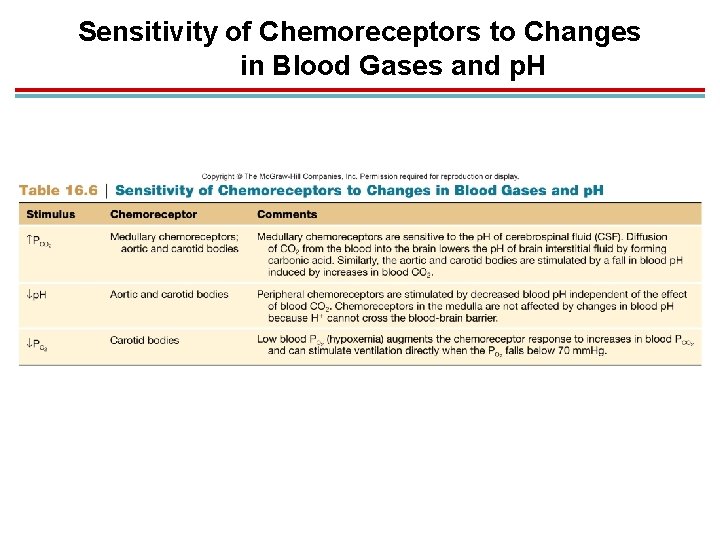
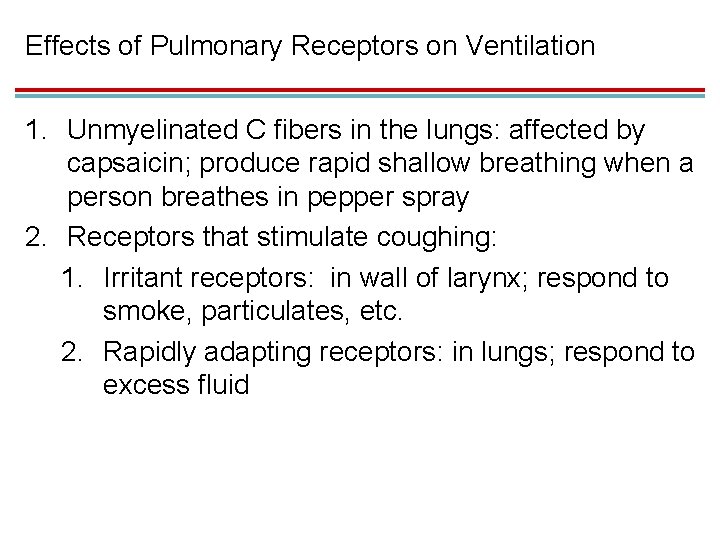
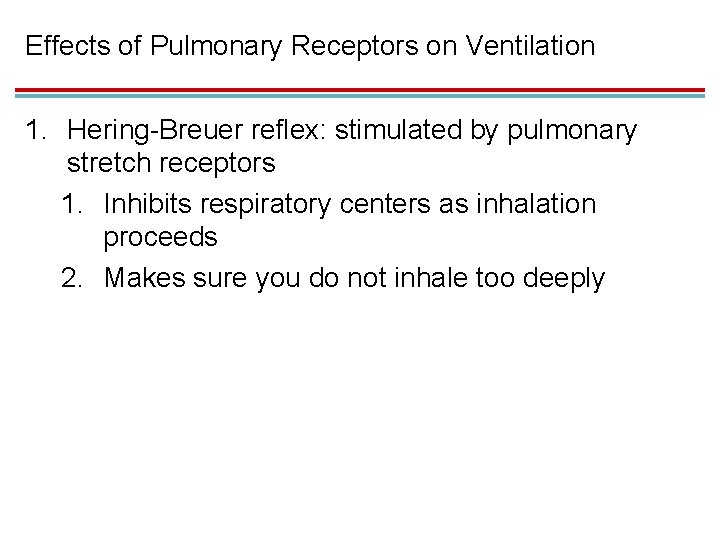
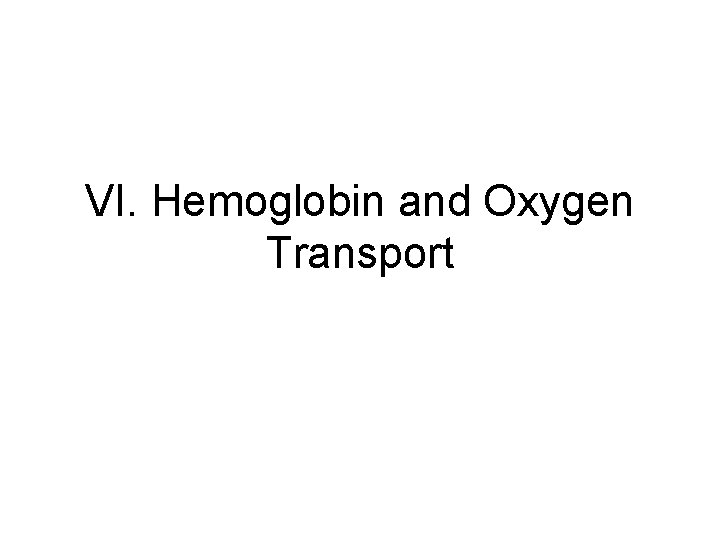
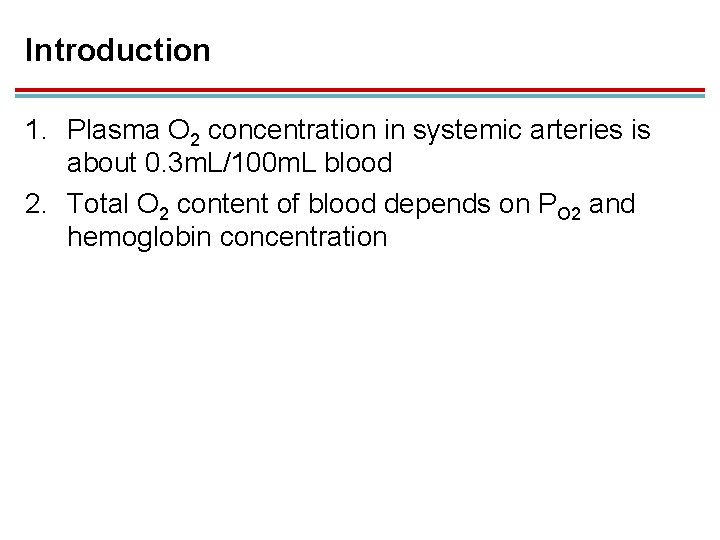
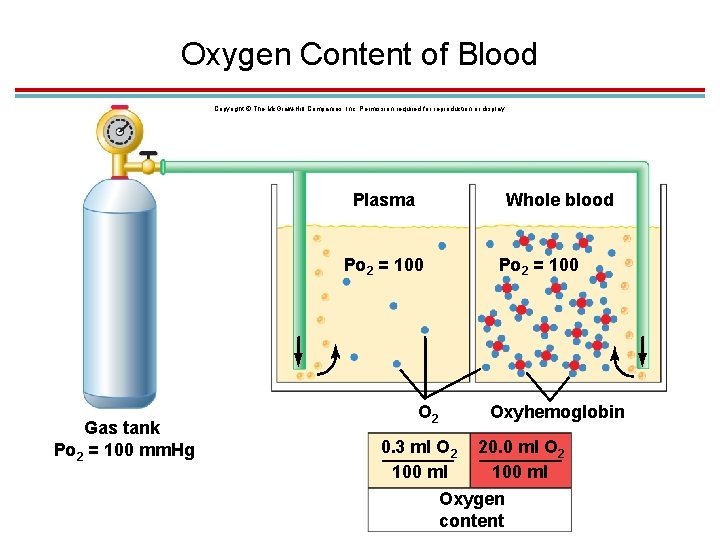
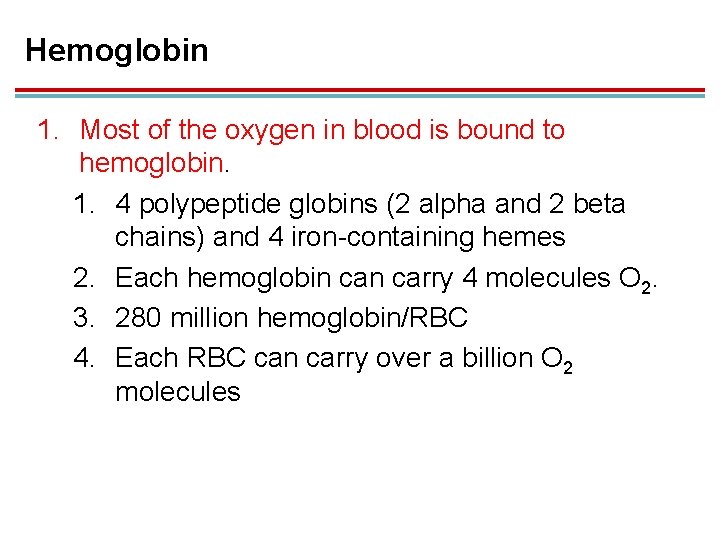
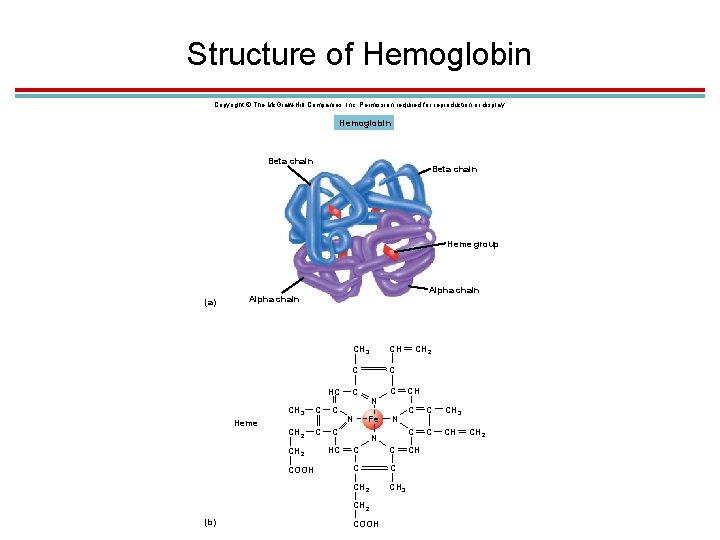
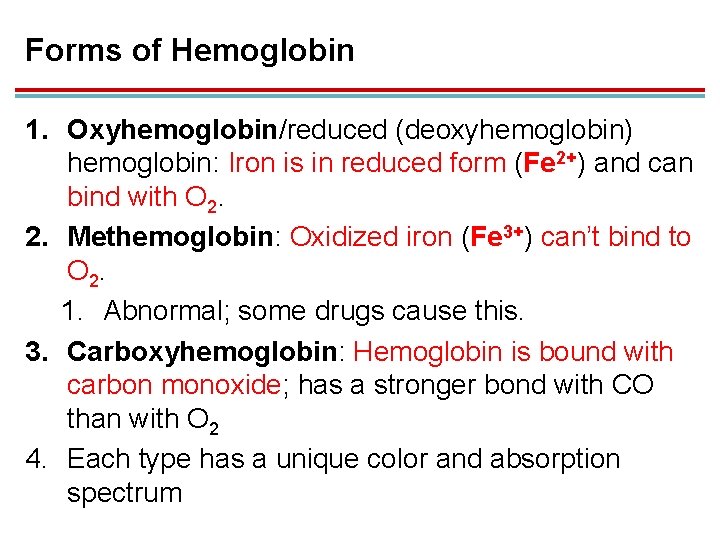
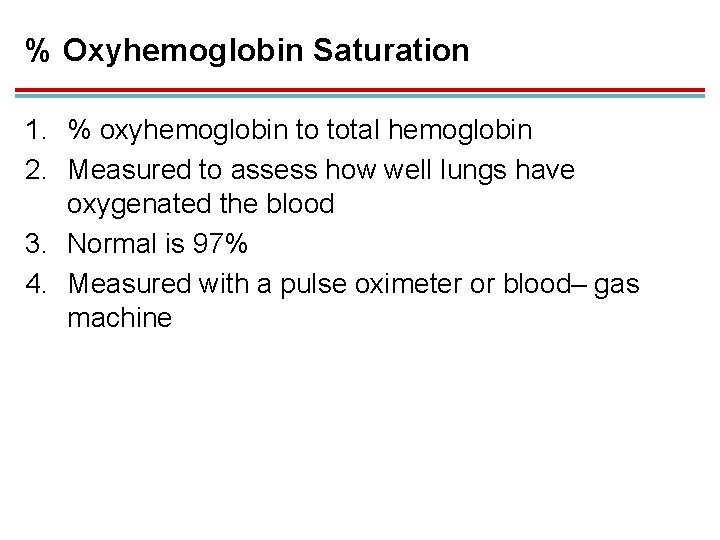
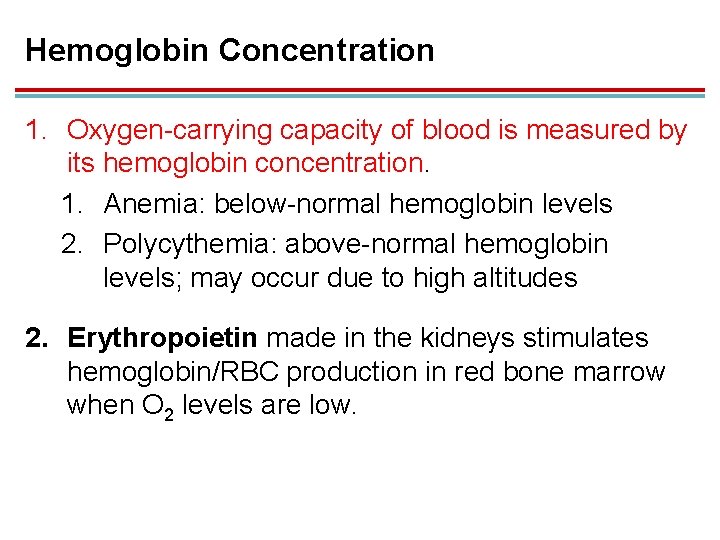
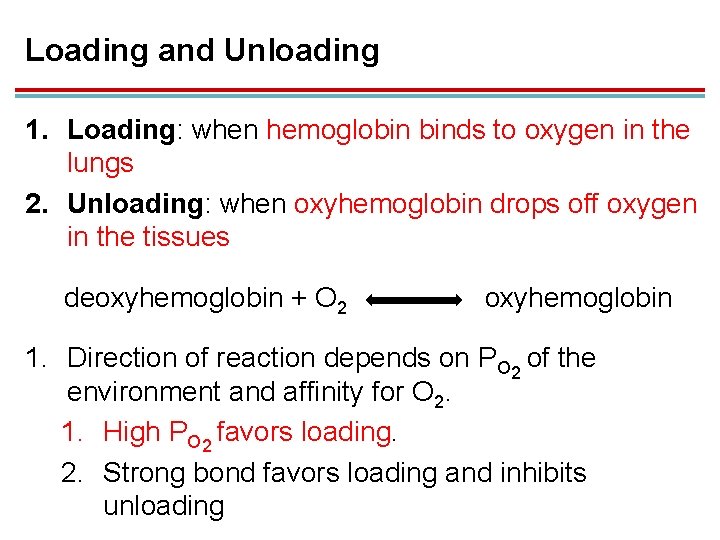
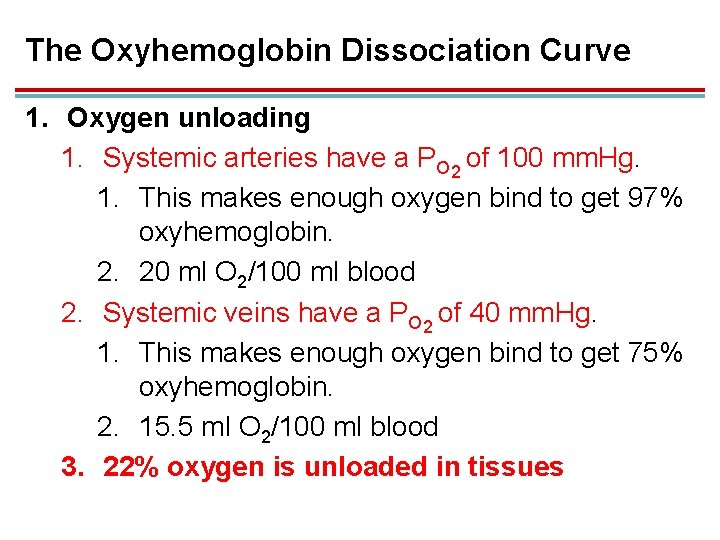
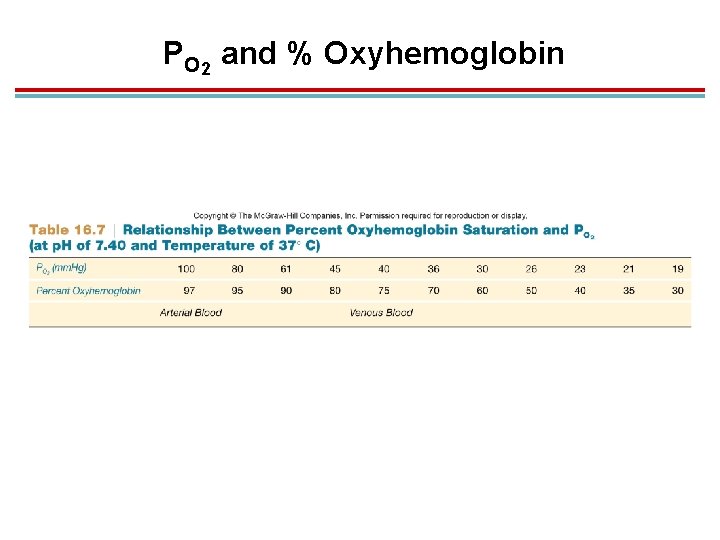
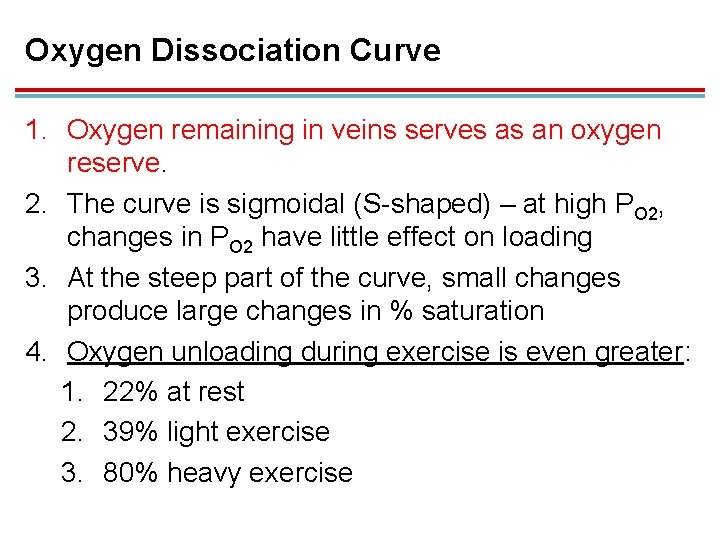
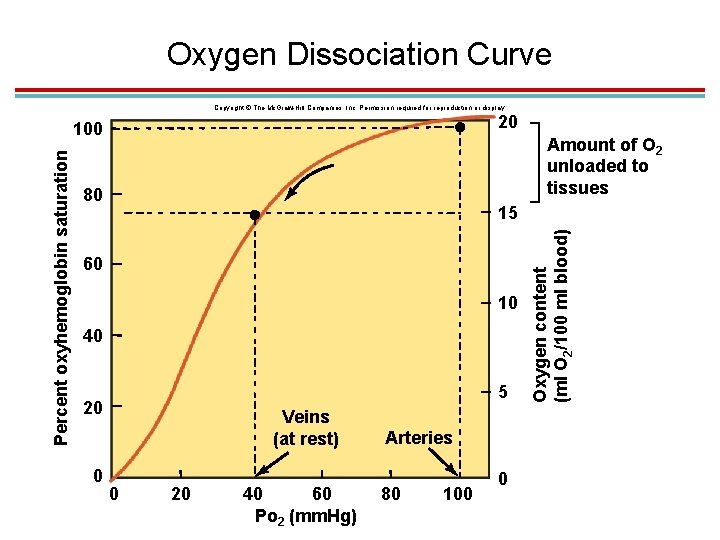
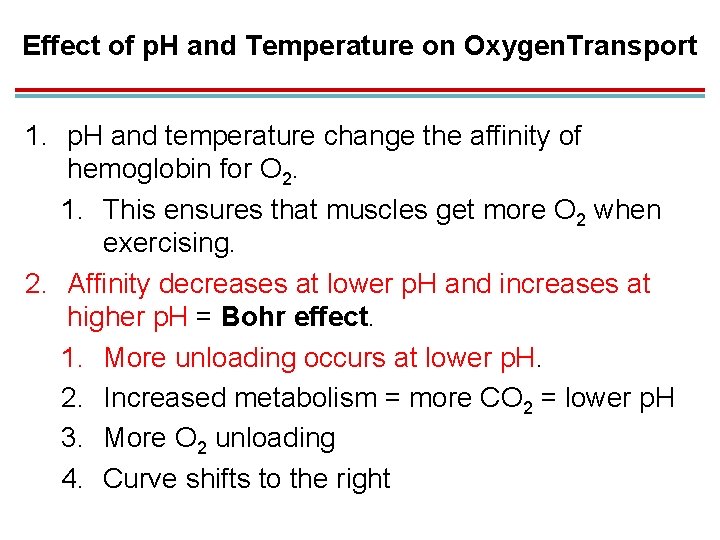
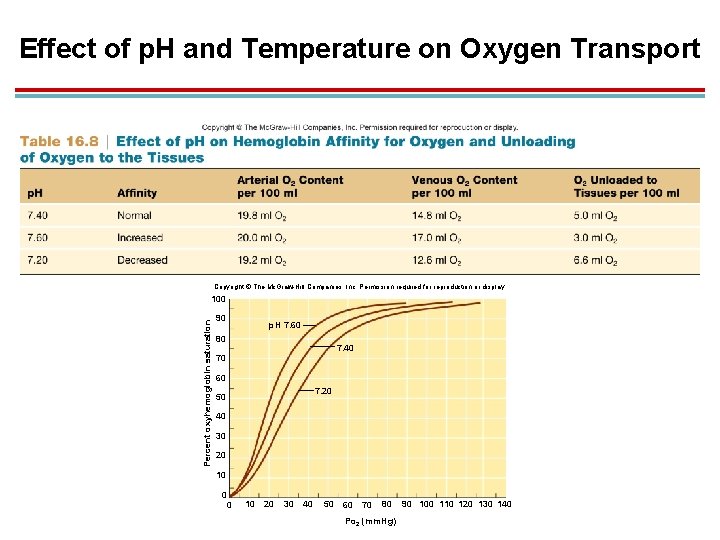
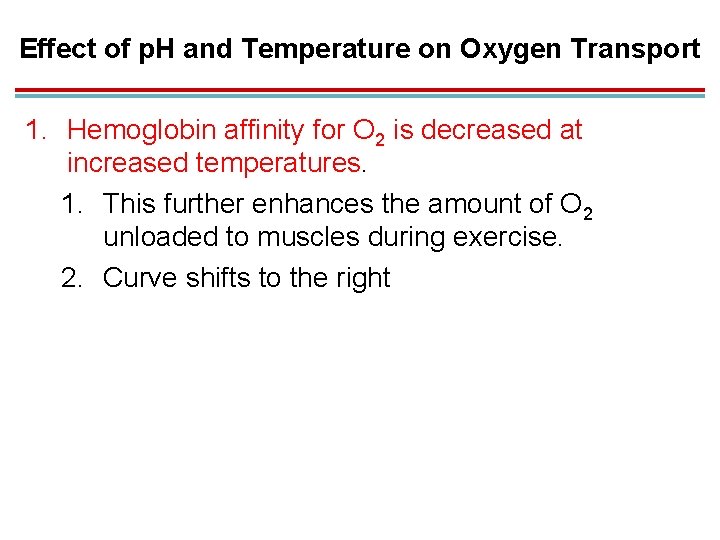
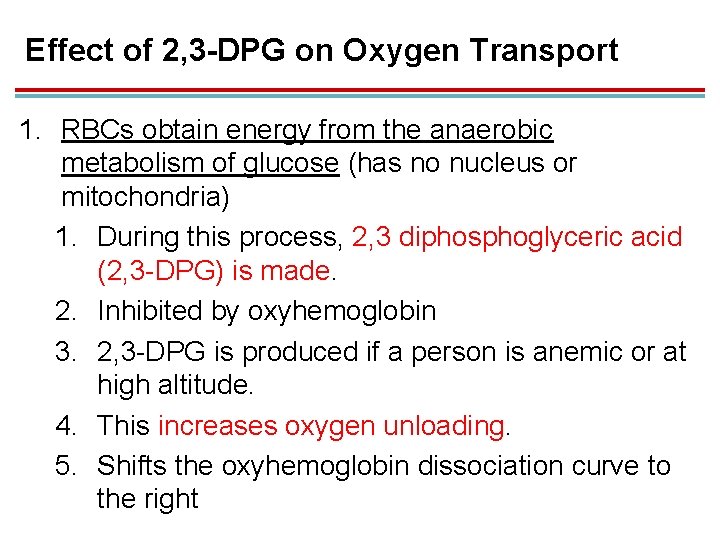
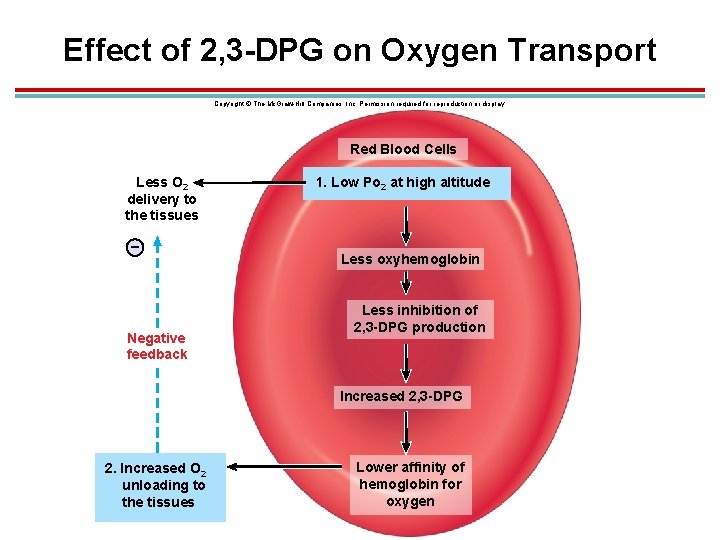
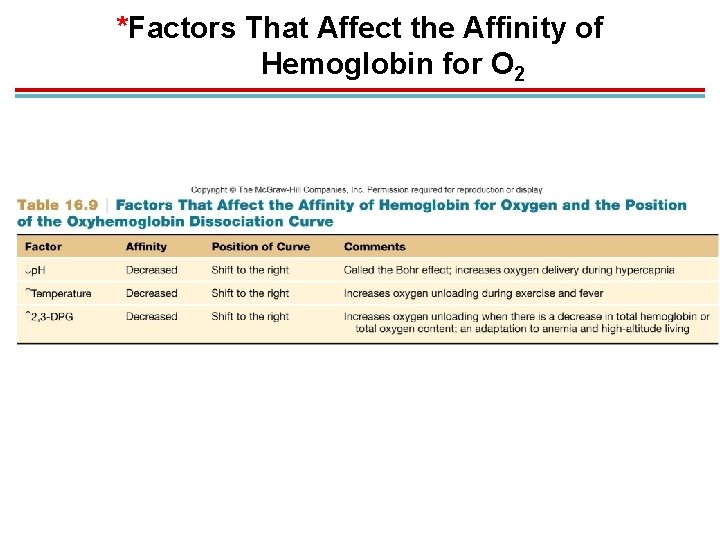
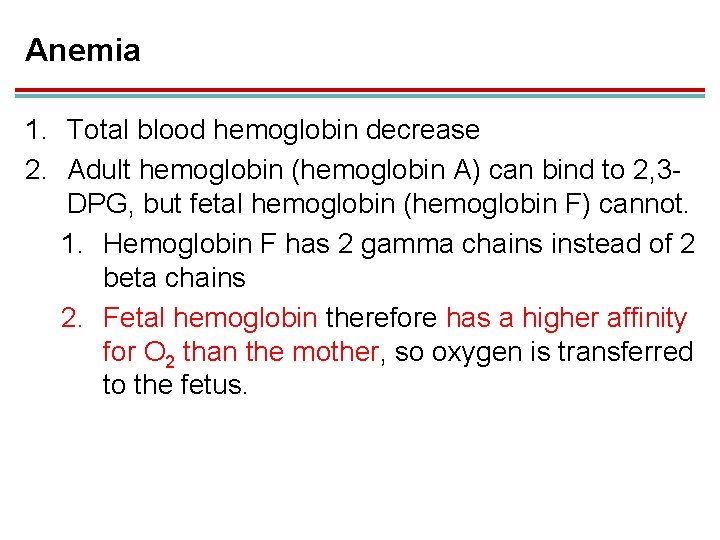
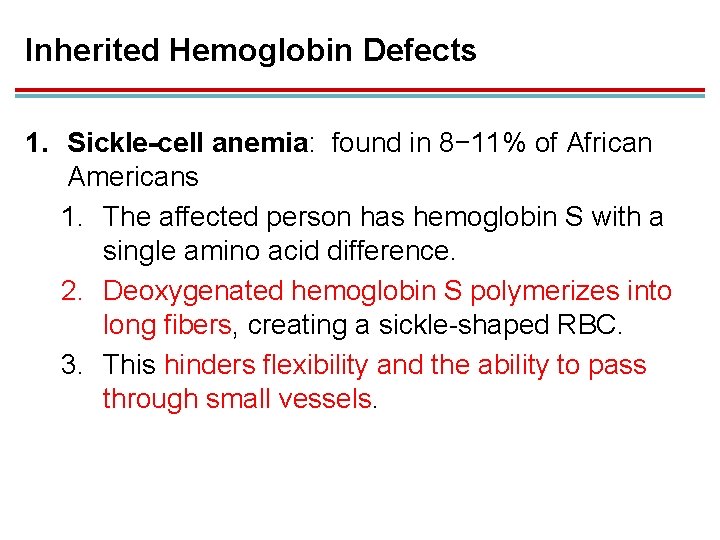
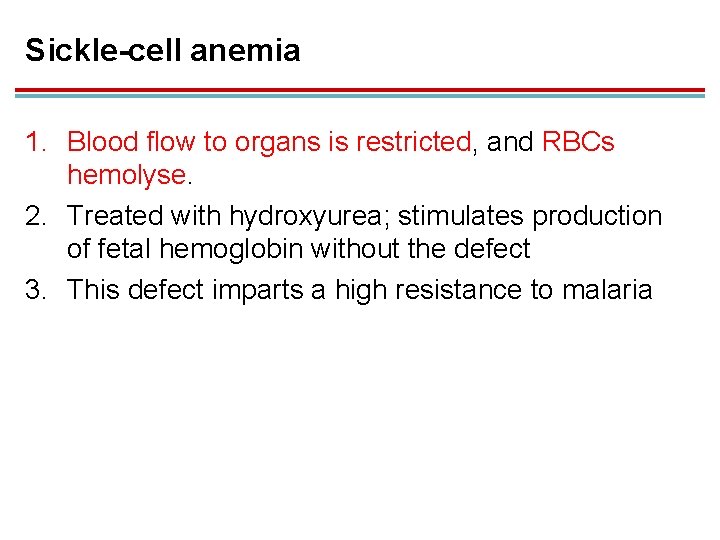
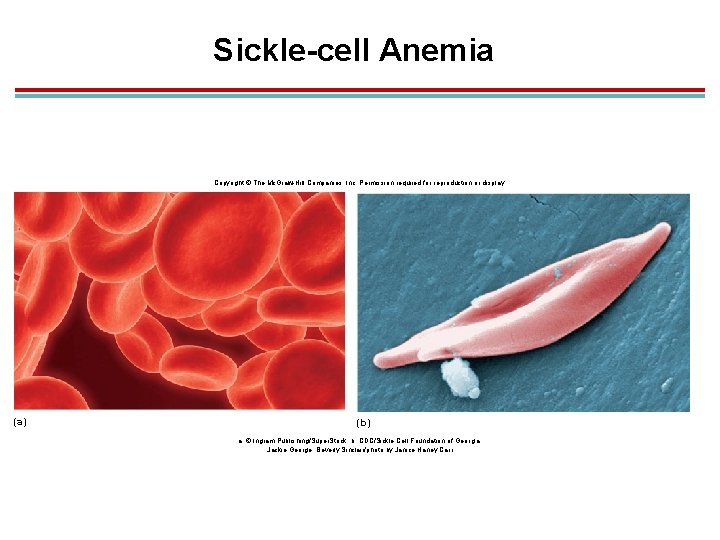
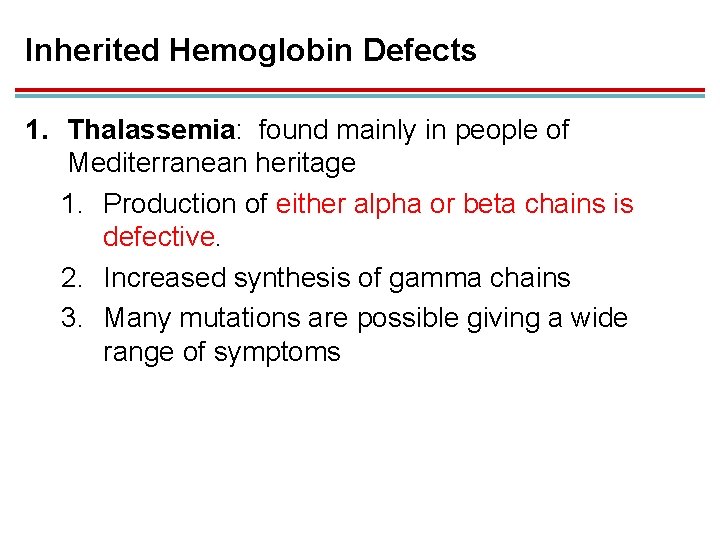
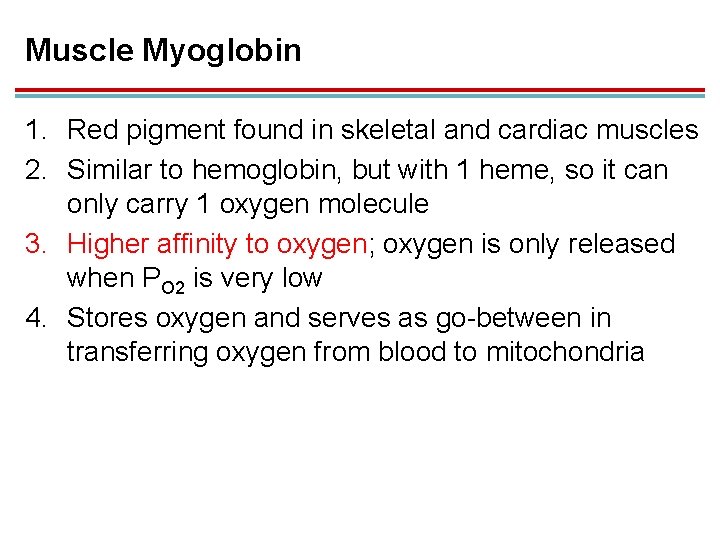
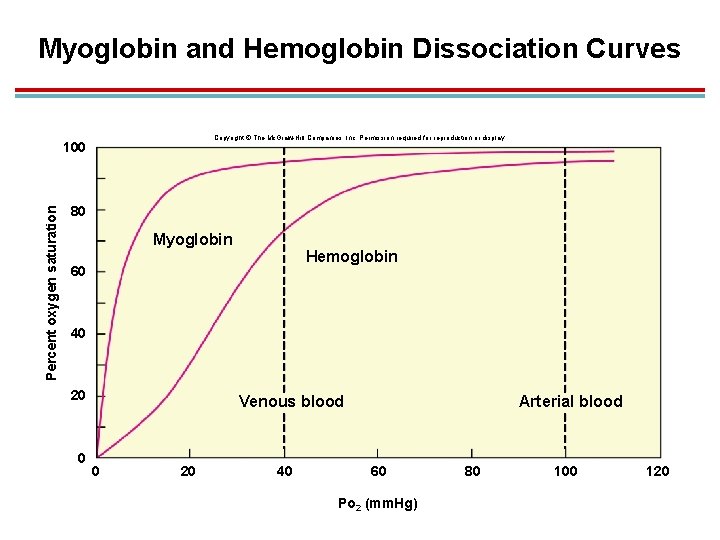
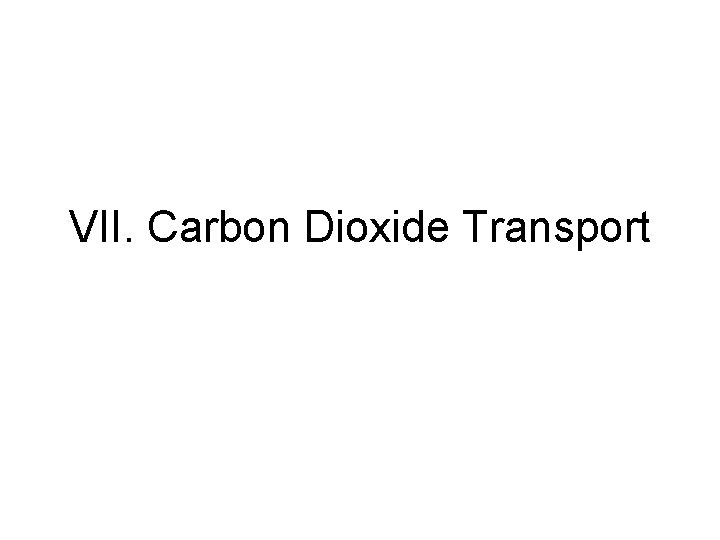
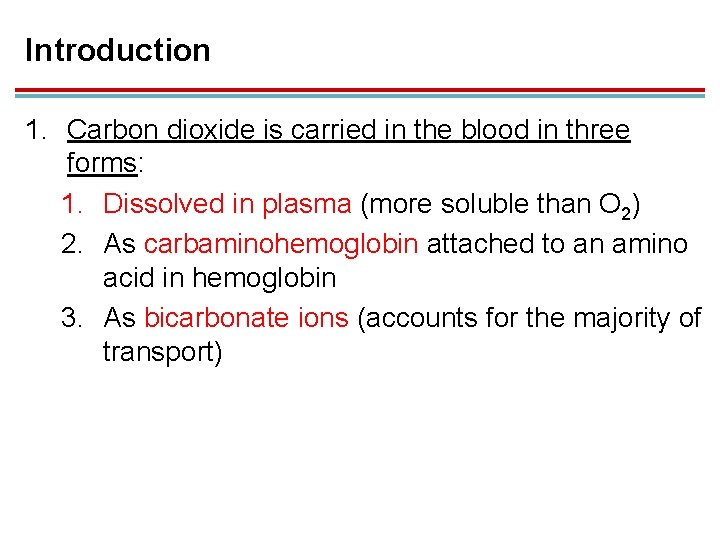
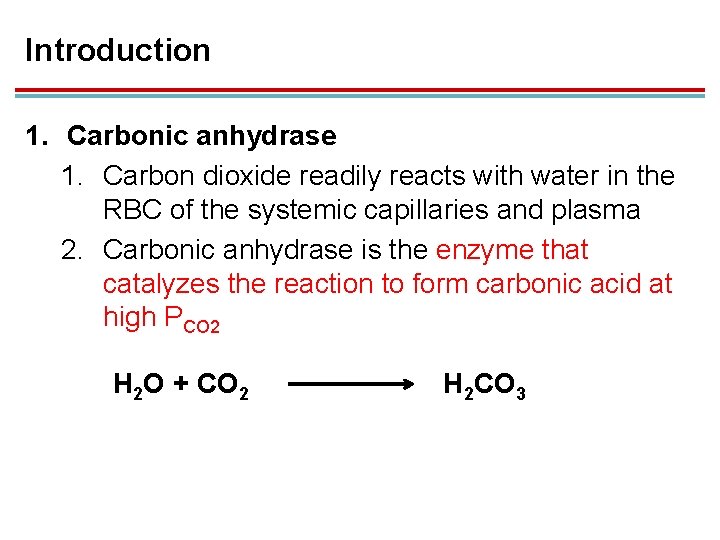
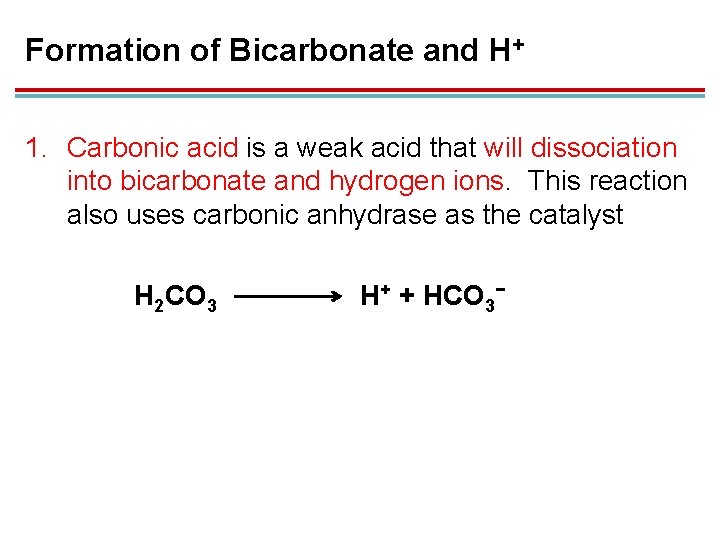
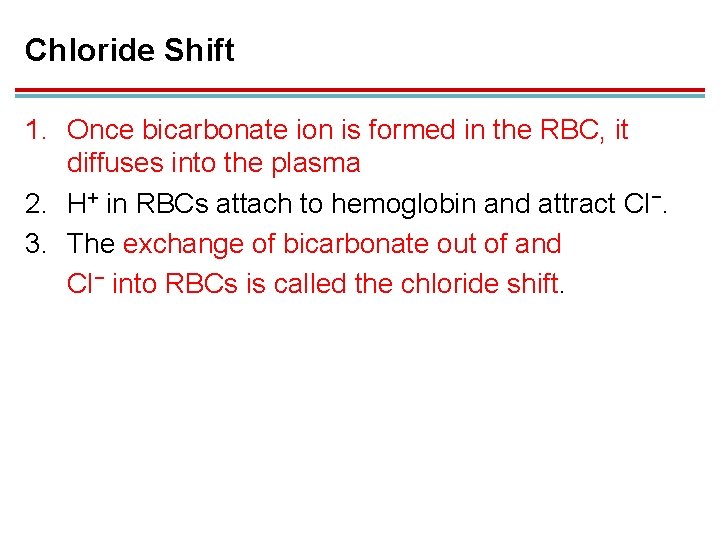
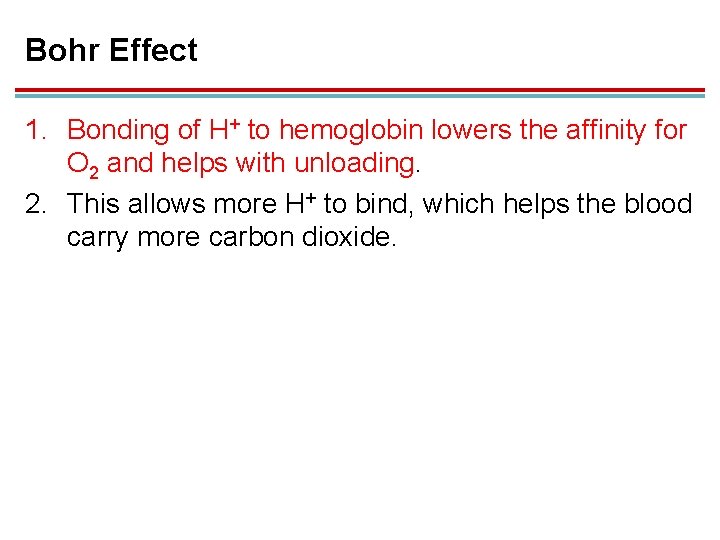
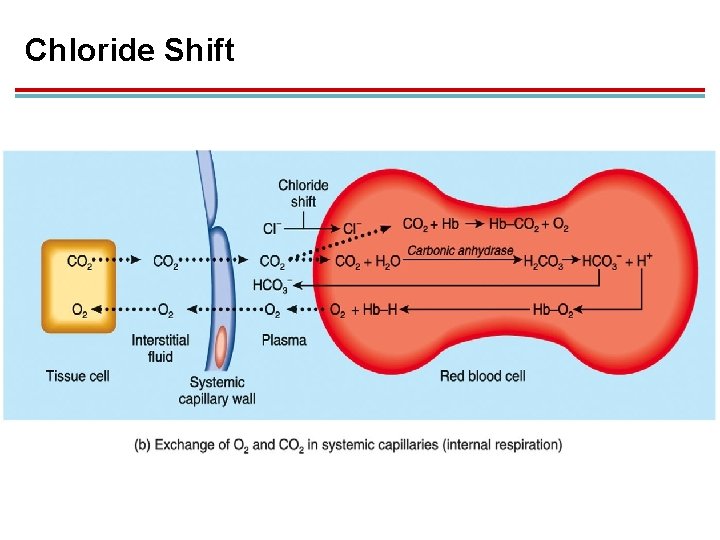
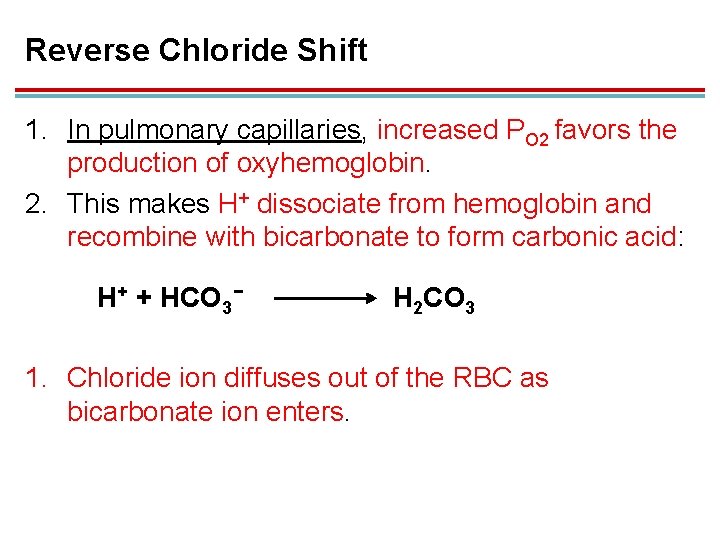
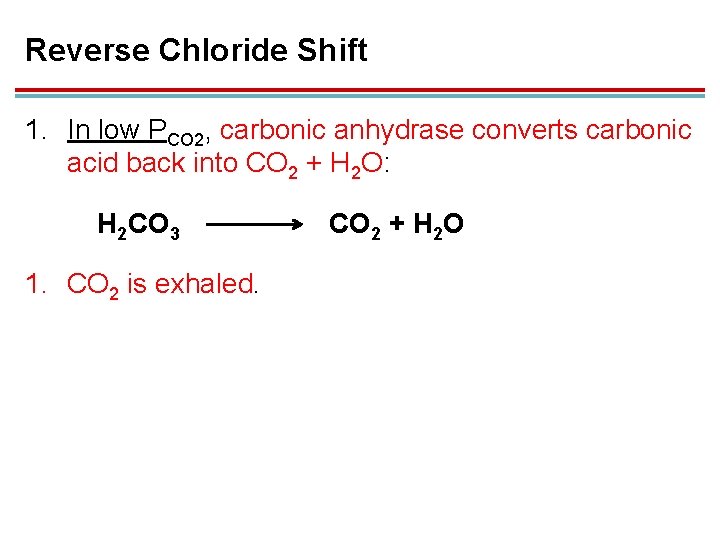
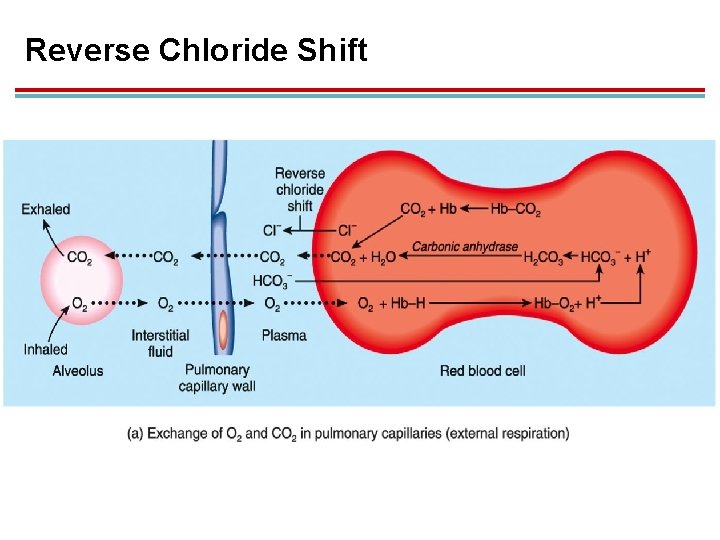
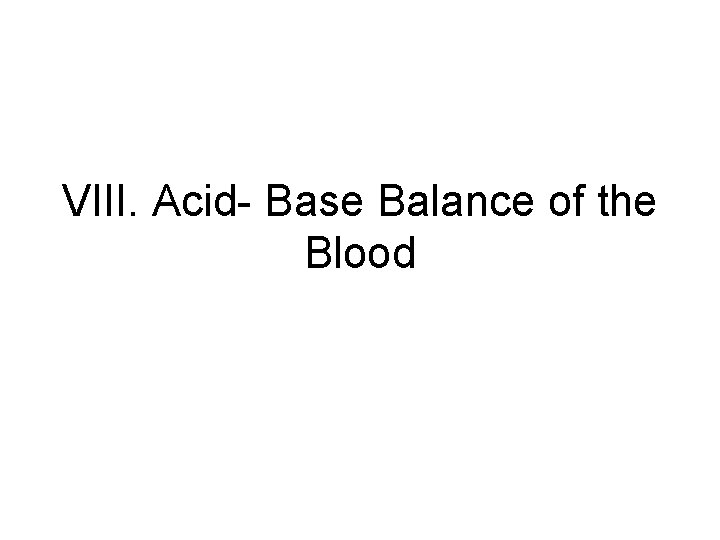
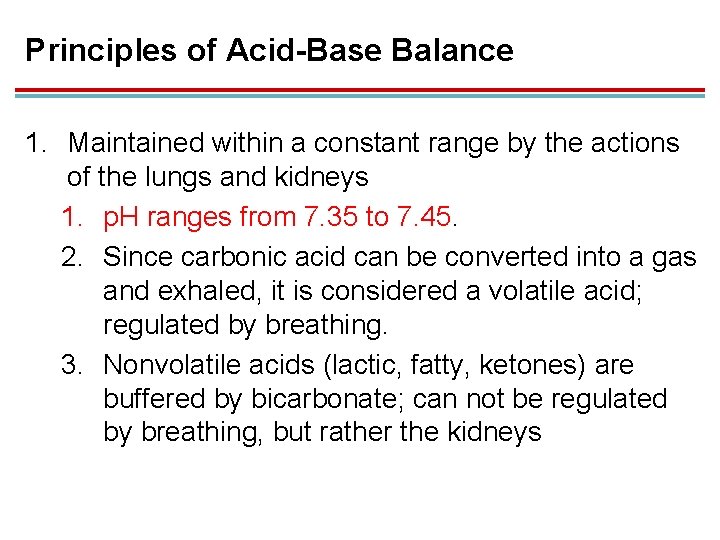
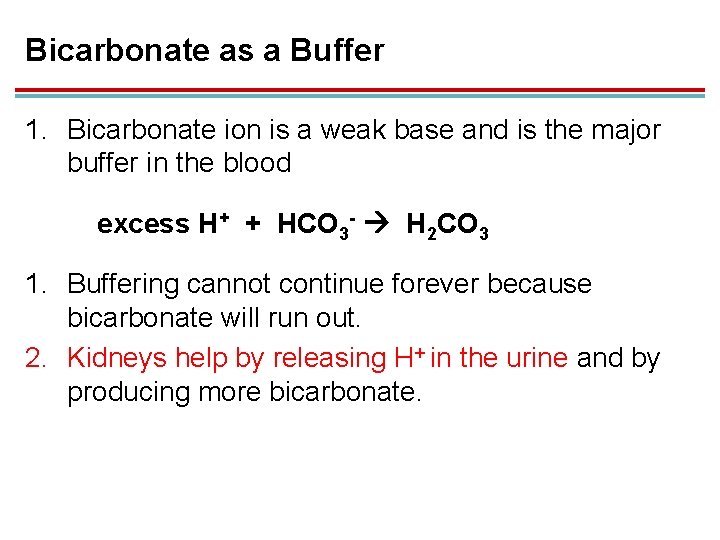
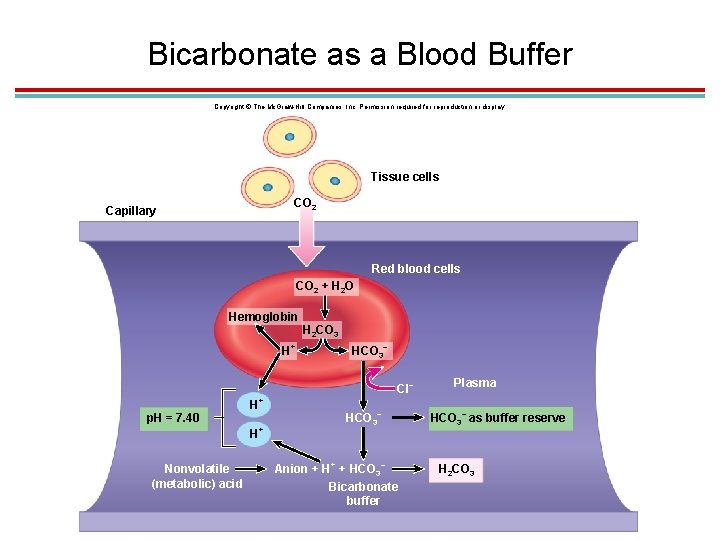
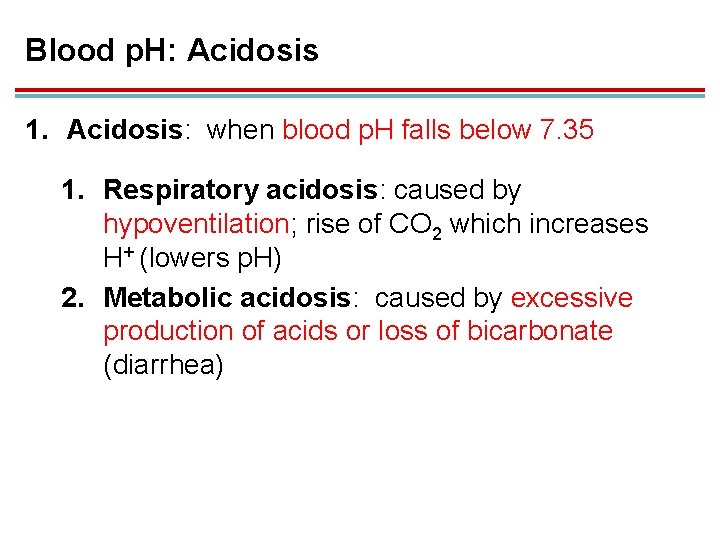
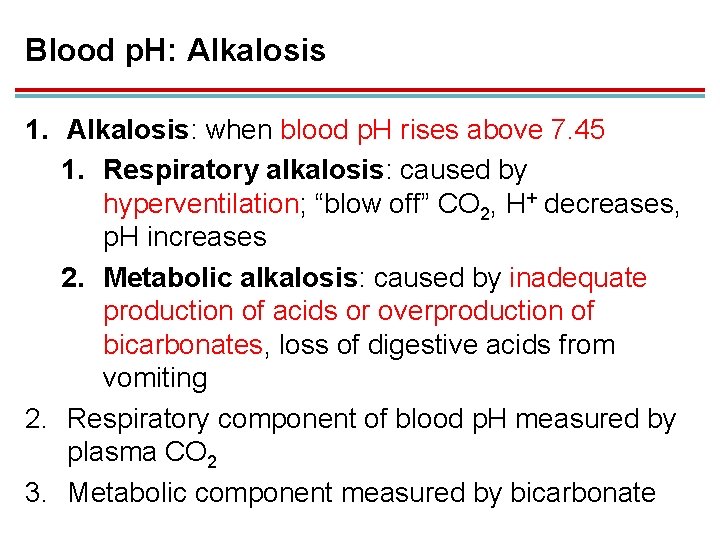
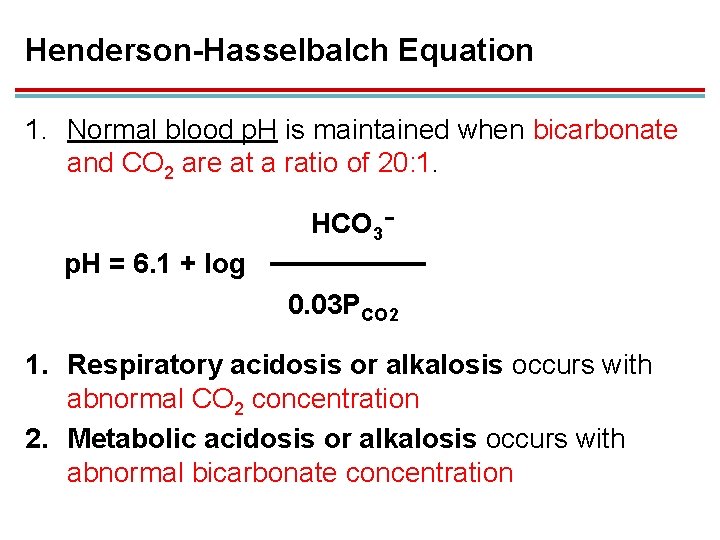
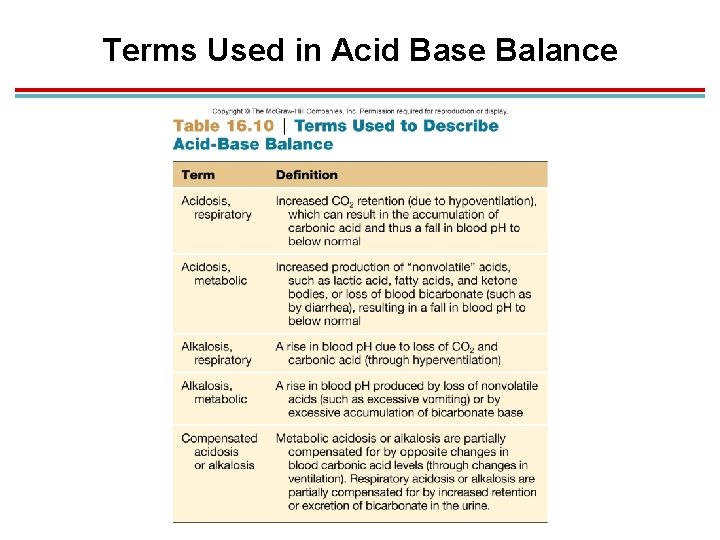
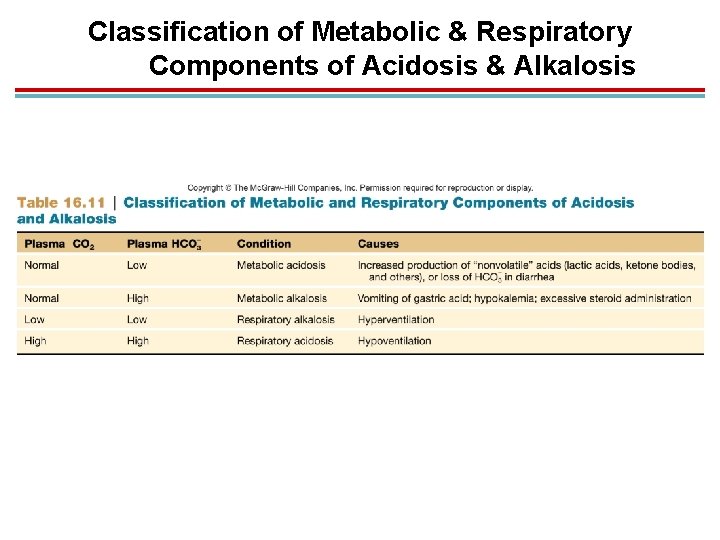
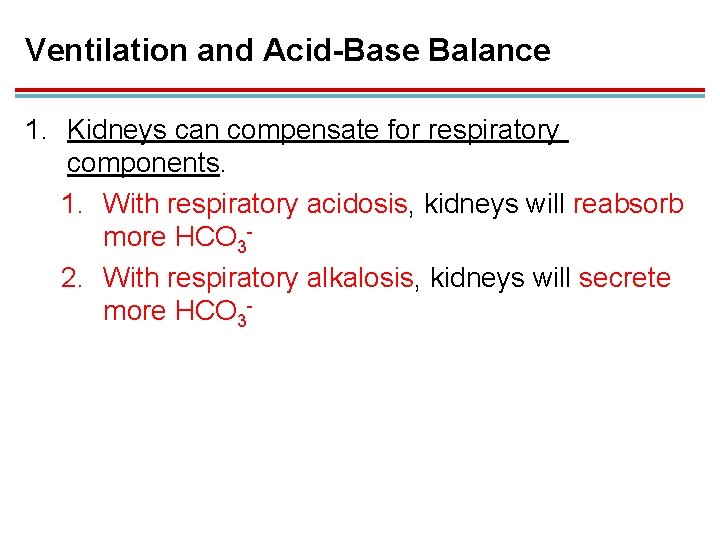
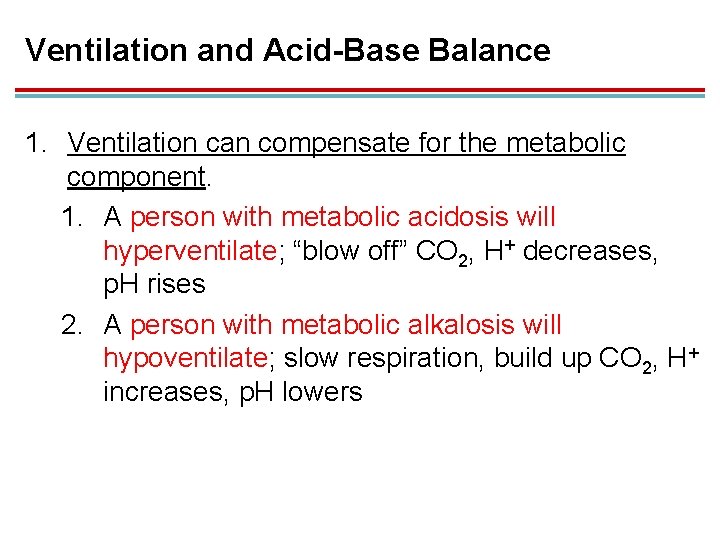
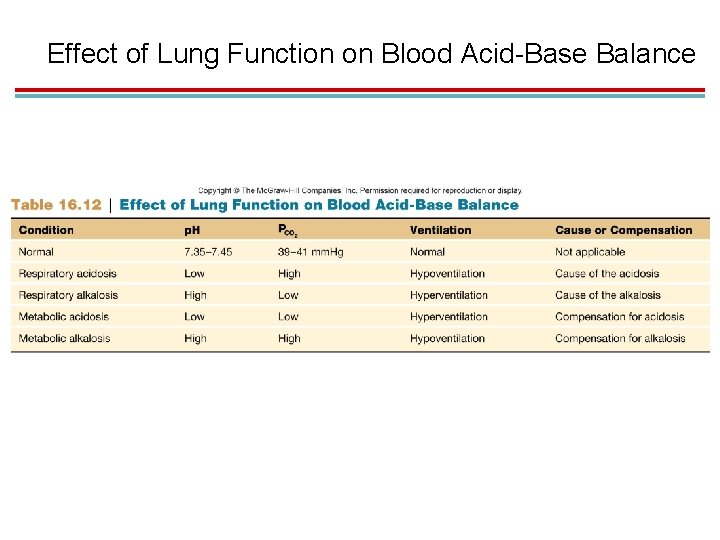
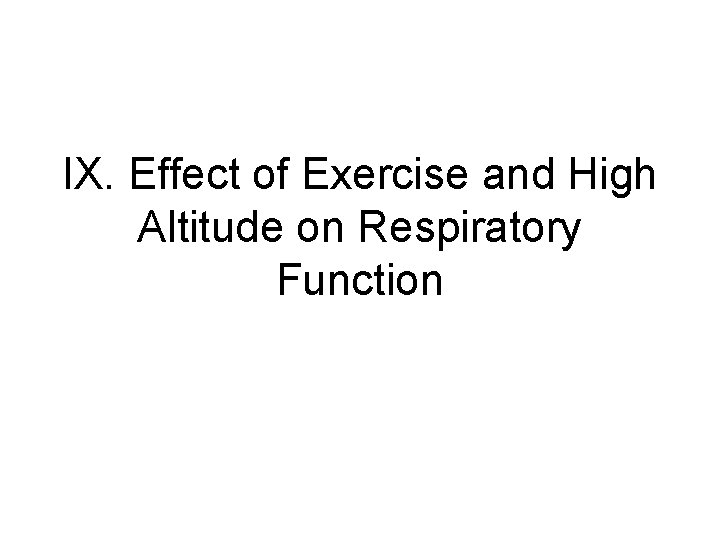
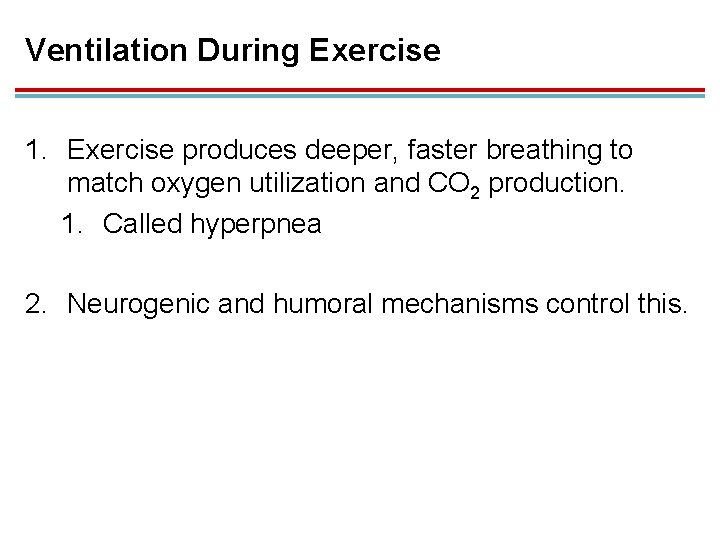
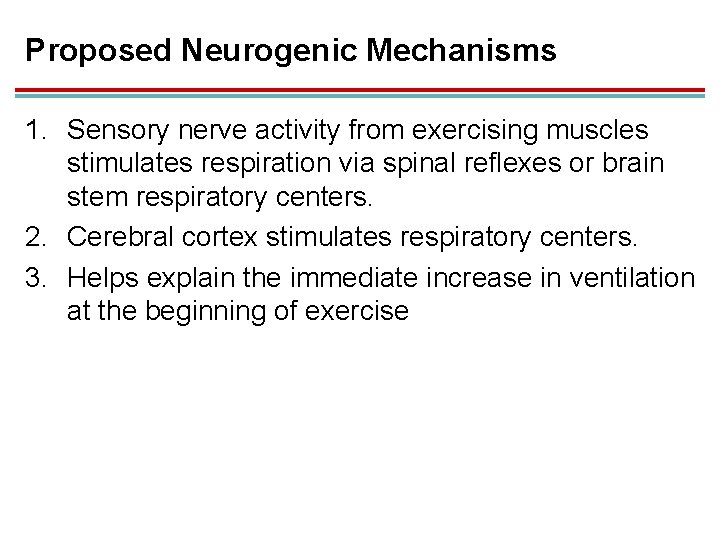
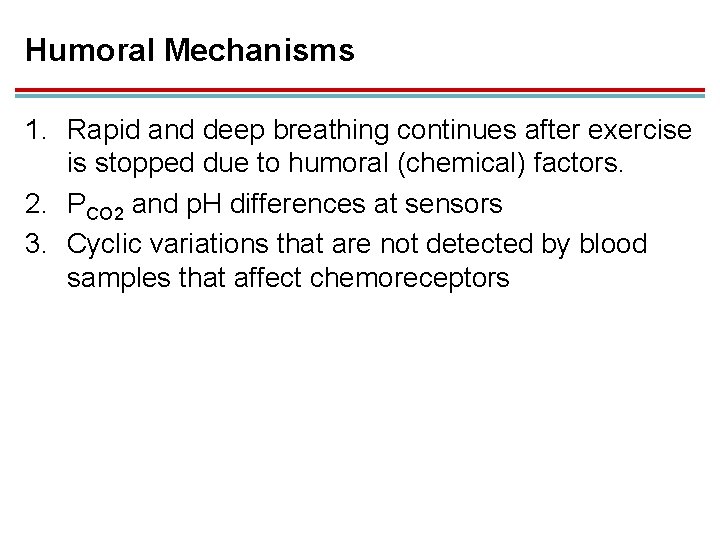
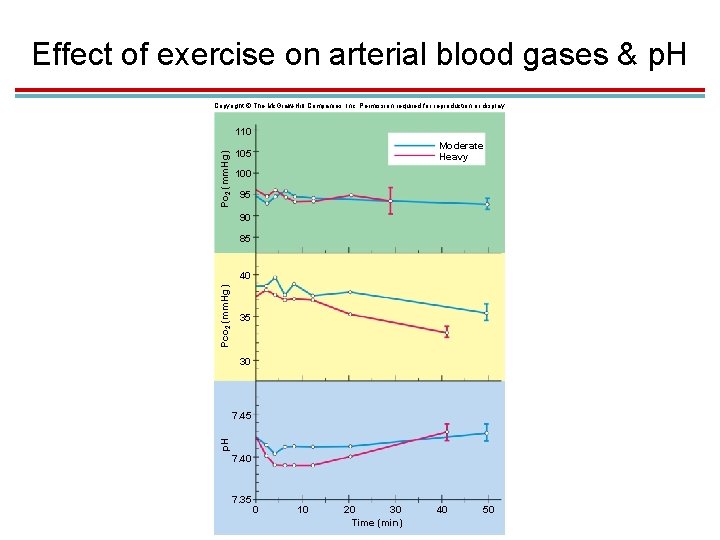
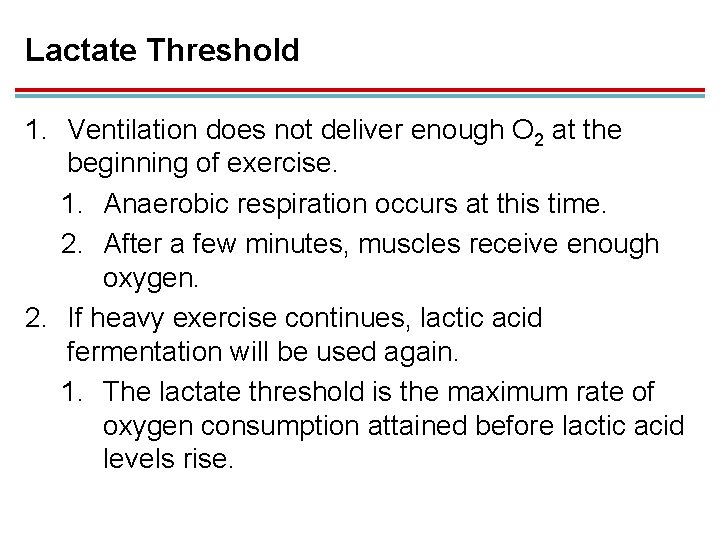
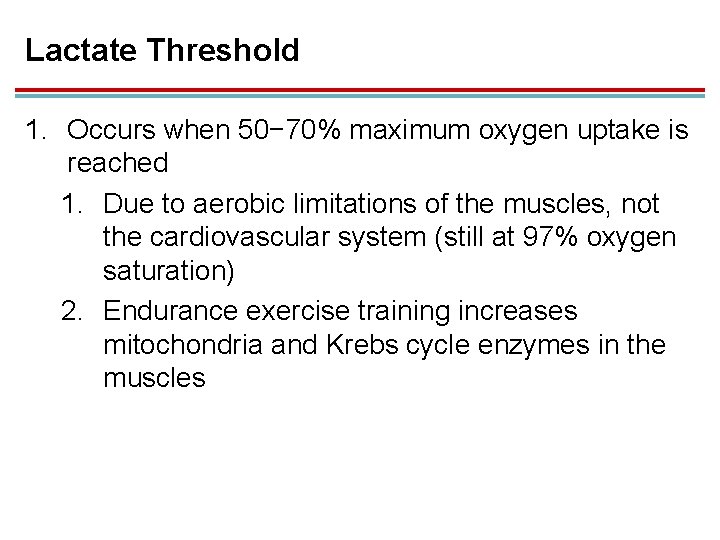
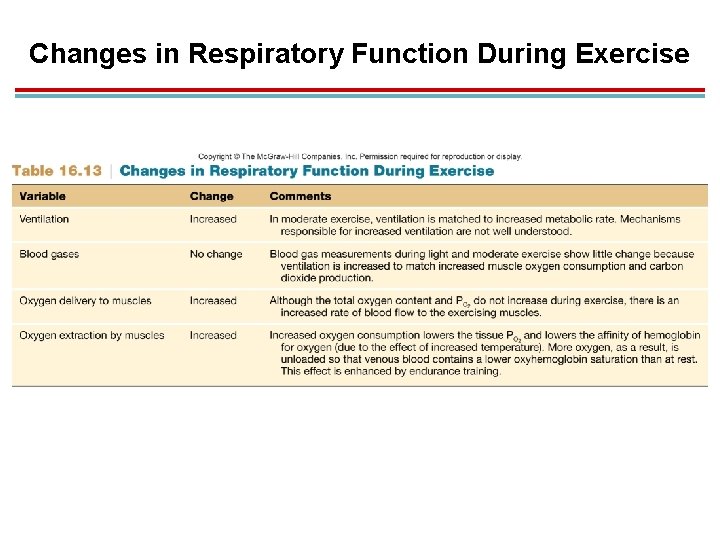
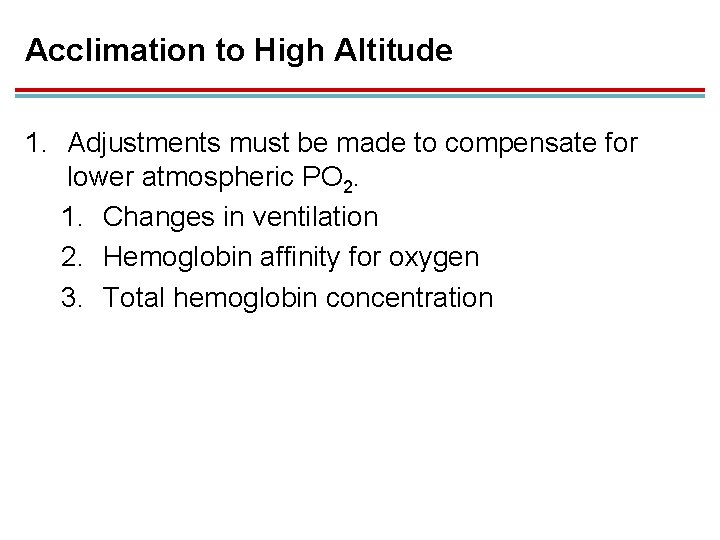
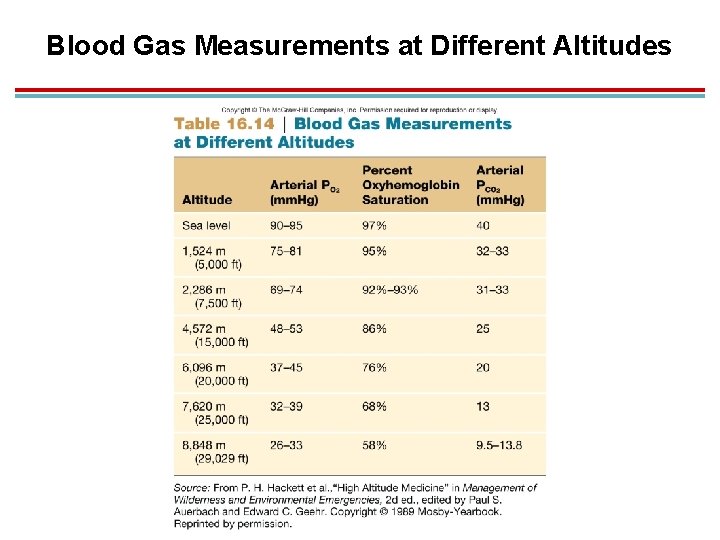
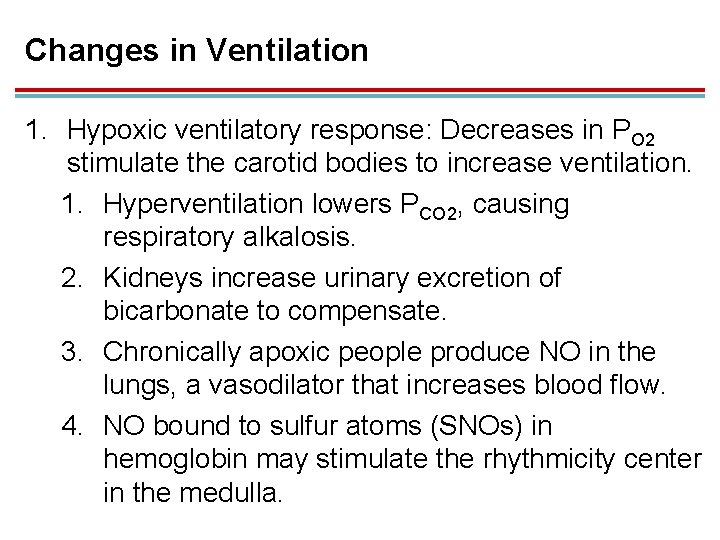
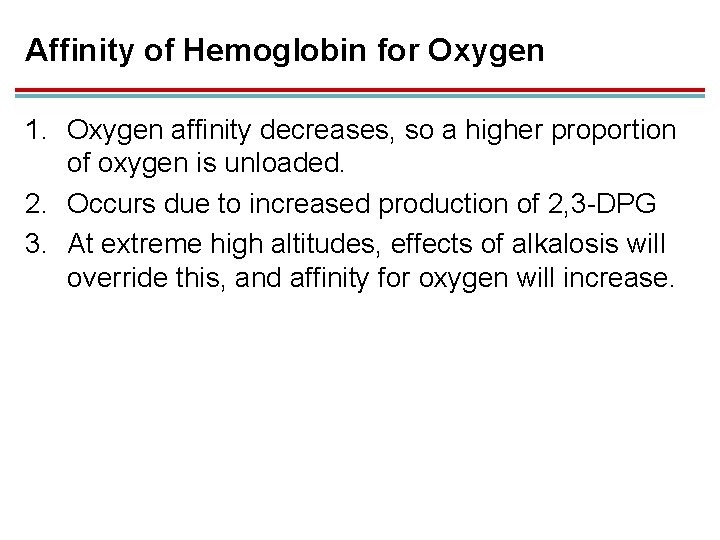
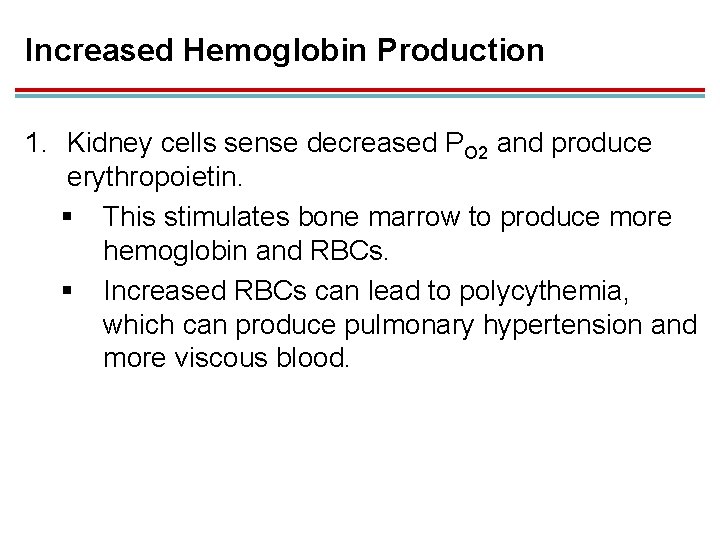
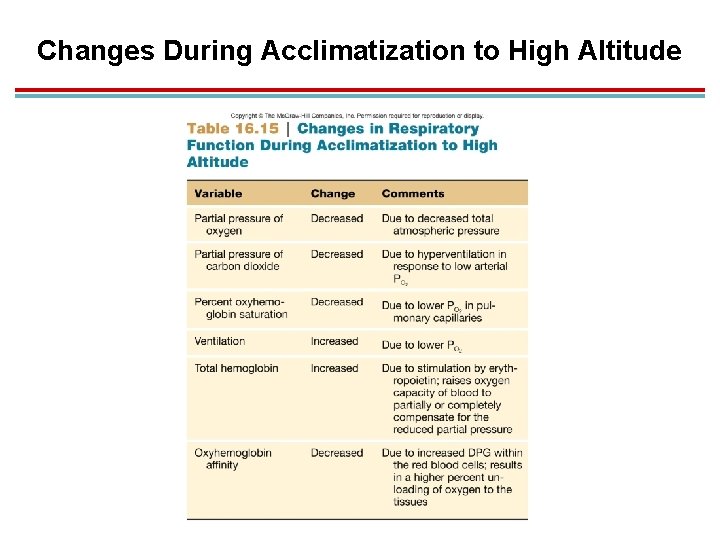
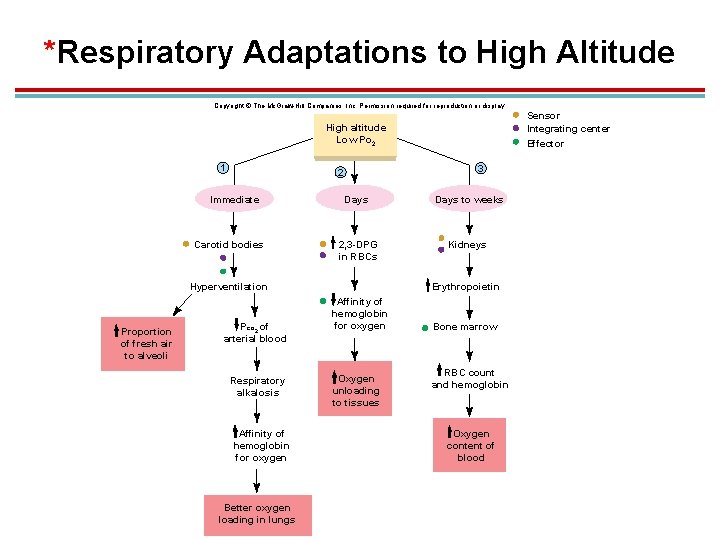
- Slides: 84
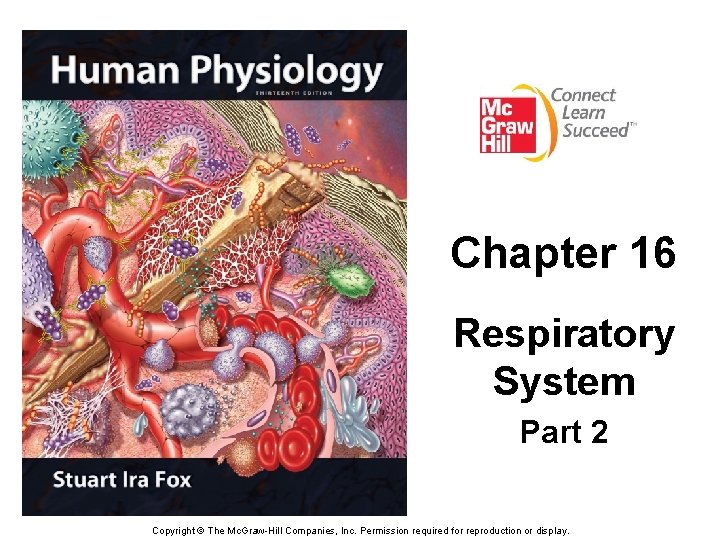
Chapter 16 Respiratory System Part 2 Copyright © The Mc. Graw-Hill Companies, Inc. Permission required for reproduction or display.
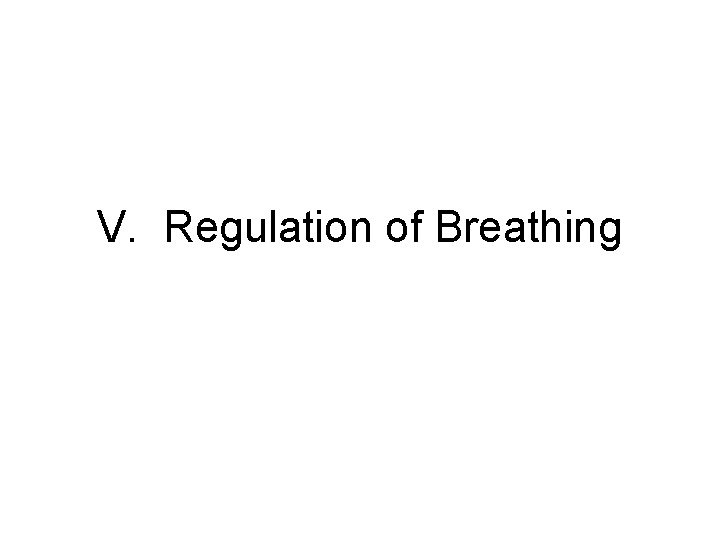
V. Regulation of Breathing
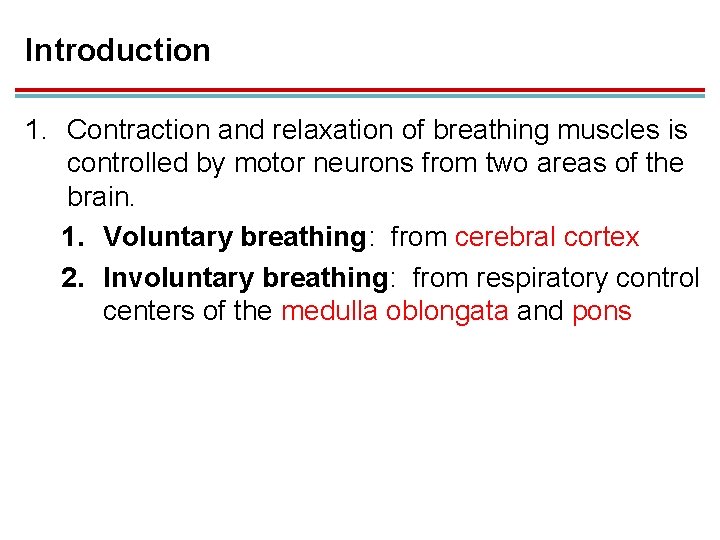
Introduction 1. Contraction and relaxation of breathing muscles is controlled by motor neurons from two areas of the brain. 1. Voluntary breathing: from cerebral cortex 2. Involuntary breathing: from respiratory control centers of the medulla oblongata and pons
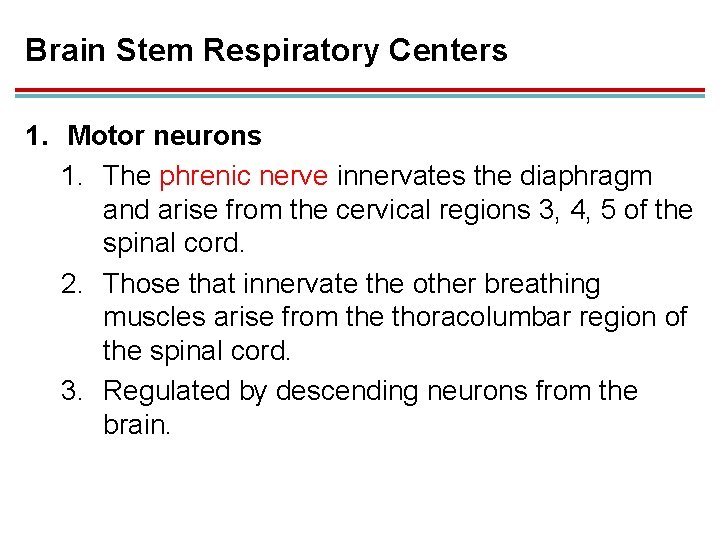
Brain Stem Respiratory Centers 1. Motor neurons 1. The phrenic nerve innervates the diaphragm and arise from the cervical regions 3, 4, 5 of the spinal cord. 2. Those that innervate the other breathing muscles arise from the thoracolumbar region of the spinal cord. 3. Regulated by descending neurons from the brain.
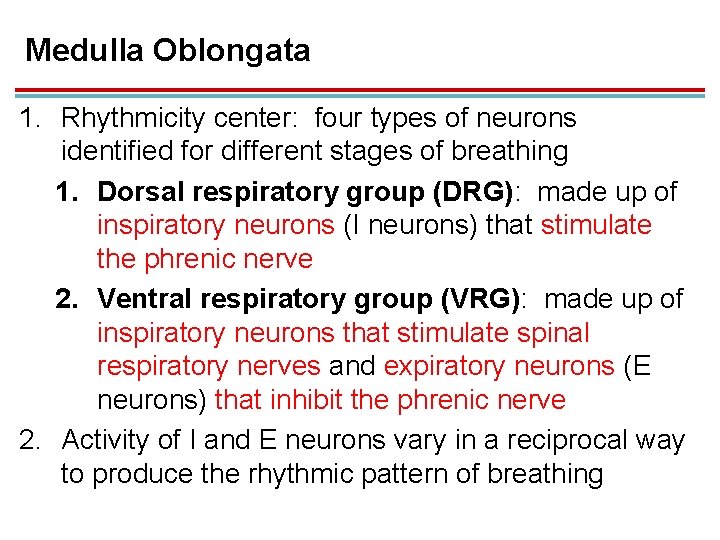
Medulla Oblongata 1. Rhythmicity center: four types of neurons identified for different stages of breathing 1. Dorsal respiratory group (DRG): made up of inspiratory neurons (I neurons) that stimulate the phrenic nerve 2. Ventral respiratory group (VRG): made up of inspiratory neurons that stimulate spinal respiratory nerves and expiratory neurons (E neurons) that inhibit the phrenic nerve 2. Activity of I and E neurons vary in a reciprocal way to produce the rhythmic pattern of breathing
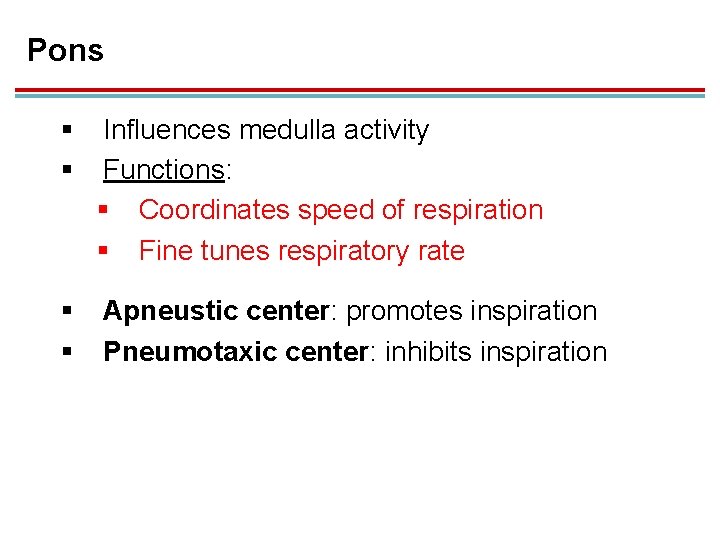
Pons § § Influences medulla activity Functions: § Coordinates speed of respiration § Fine tunes respiratory rate § § Apneustic center: promotes inspiration Pneumotaxic center: inhibits inspiration
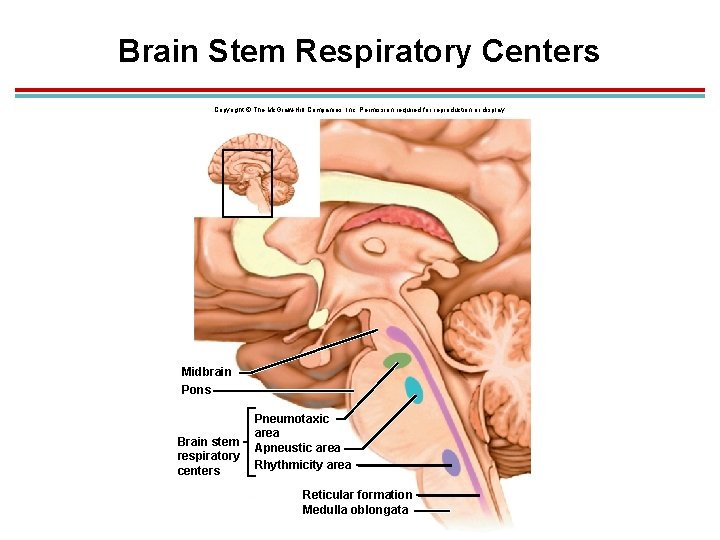
Brain Stem Respiratory Centers Copyright © The Mc. Graw-Hill Companies, Inc. Permission required for reproduction or display. Midbrain Pons Brain stem respiratory centers Pneumotaxic area Apneustic area Rhythmicity area Reticular formation Medulla oblongata
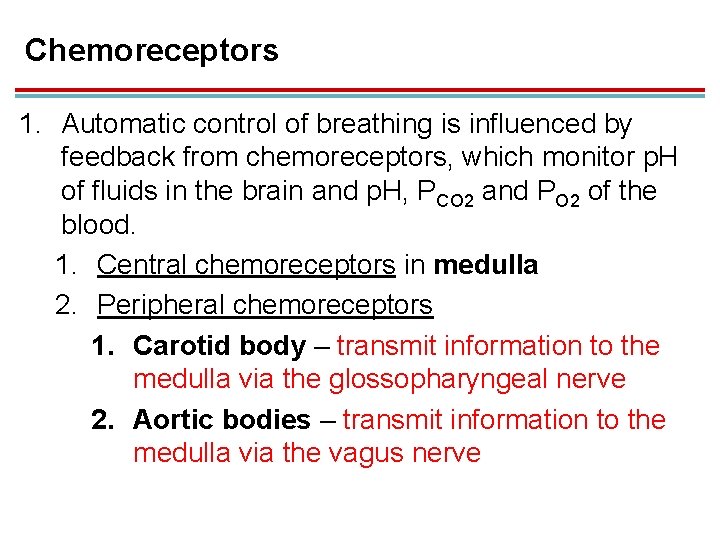
Chemoreceptors 1. Automatic control of breathing is influenced by feedback from chemoreceptors, which monitor p. H of fluids in the brain and p. H, PCO 2 and PO 2 of the blood. 1. Central chemoreceptors in medulla 2. Peripheral chemoreceptors 1. Carotid body – transmit information to the medulla via the glossopharyngeal nerve 2. Aortic bodies – transmit information to the medulla via the vagus nerve
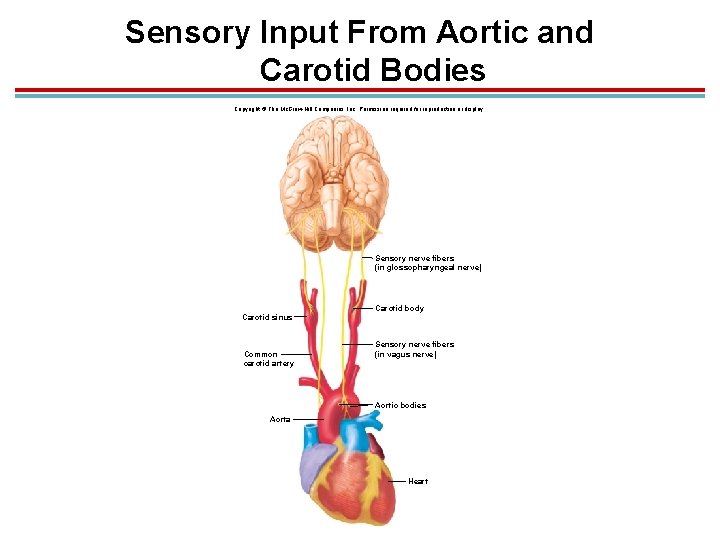
Sensory Input From Aortic and Carotid Bodies Copyright © The Mc. Graw-Hill Companies, Inc. Permission required for reproduction or display. Sensory nerve fibers (in glossopharyngeal nerve) Carotid body Carotid sinus Common carotid artery Sensory nerve fibers (in vagus nerve) Aortic bodies Aorta Heart
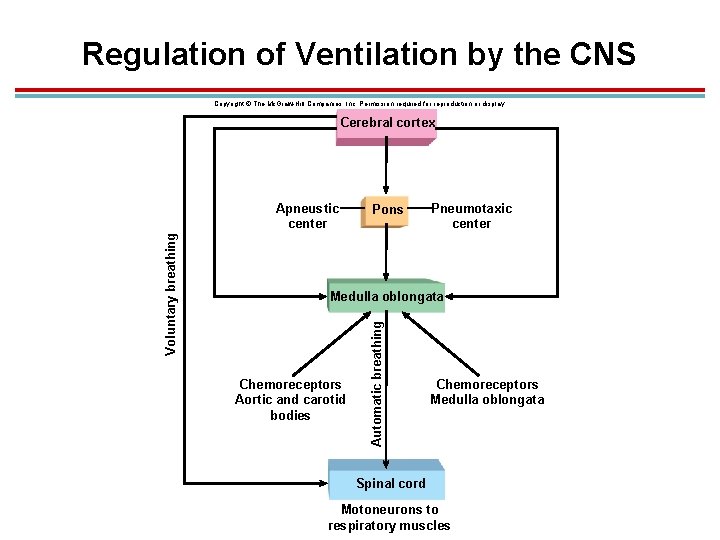
Regulation of Ventilation by the CNS Copyright © The Mc. Graw-Hill Companies, Inc. Permission required for reproduction or display. Cerebral cortex Pons Pneumotaxic center Medulla oblongata Chemoreceptors Aortic and carotid bodies Automatic breathing Voluntary breathing Apneustic center Chemoreceptors Medulla oblongata Spinal cord Motoneurons to respiratory muscles
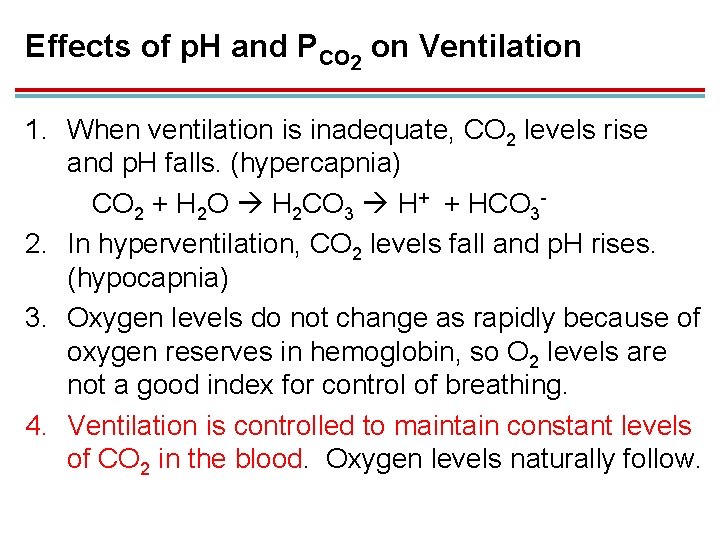
Effects of p. H and PCO 2 on Ventilation 1. When ventilation is inadequate, CO 2 levels rise and p. H falls. (hypercapnia) CO 2 + H 2 O H 2 CO 3 H+ + HCO 32. In hyperventilation, CO 2 levels fall and p. H rises. (hypocapnia) 3. Oxygen levels do not change as rapidly because of oxygen reserves in hemoglobin, so O 2 levels are not a good index for control of breathing. 4. Ventilation is controlled to maintain constant levels of CO 2 in the blood. Oxygen levels naturally follow.
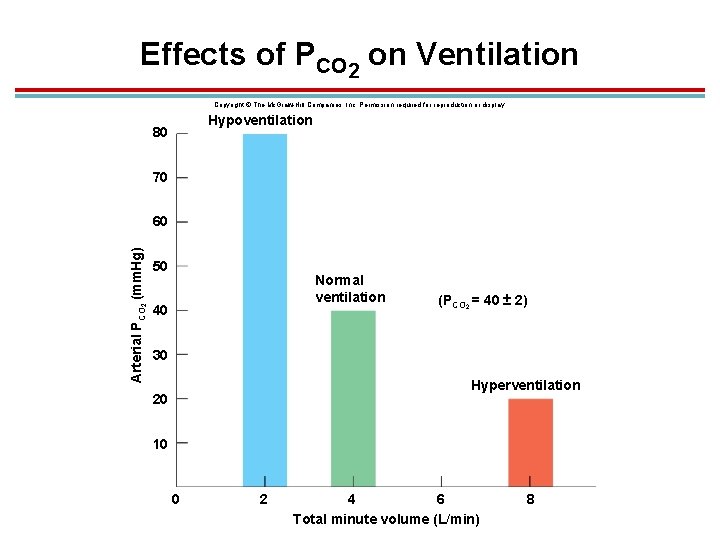
Effects of PCO 2 on Ventilation Copyright © The Mc. Graw-Hill Companies, Inc. Permission required for reproduction or display. Hypoventilation 80 70 Arterial PCO 2 (mm. Hg) 60 50 Normal ventilation 40 (PCO 2 = 40 ± 2) 30 Hyperventilation 20 10 0 2 4 6 Total minute volume (L/min) 8
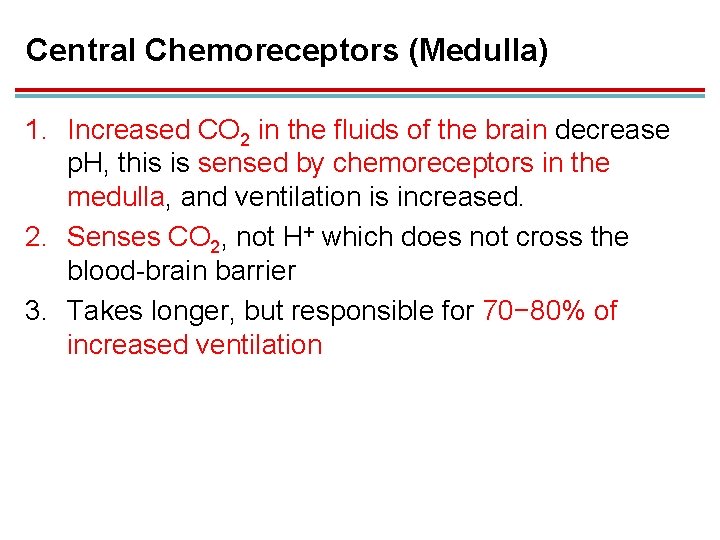
Central Chemoreceptors (Medulla) 1. Increased CO 2 in the fluids of the brain decrease p. H, this is sensed by chemoreceptors in the medulla, and ventilation is increased. 2. Senses CO 2, not H+ which does not cross the blood-brain barrier 3. Takes longer, but responsible for 70− 80% of increased ventilation
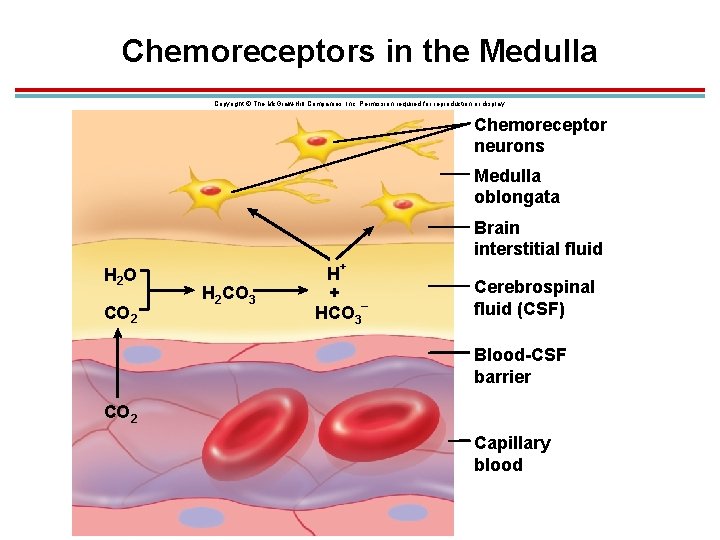
Chemoreceptors in the Medulla Copyright © The Mc. Graw-Hill Companies, Inc. Permission required for reproduction or display. Chemoreceptor neurons Medulla oblongata Brain interstitial fluid H 2 O CO 2 + H 2 CO 3 H + – HCO 3 Cerebrospinal fluid (CSF) Blood-CSF barrier CO 2 Capillary blood
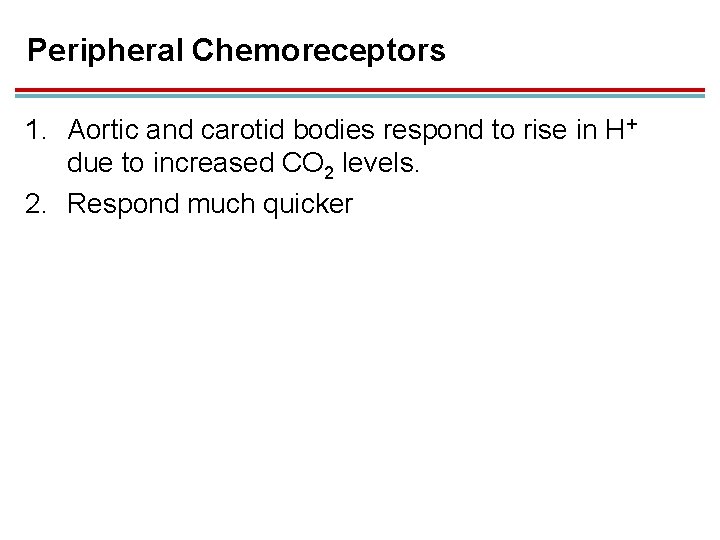
Peripheral Chemoreceptors 1. Aortic and carotid bodies respond to rise in H+ due to increased CO 2 levels. 2. Respond much quicker
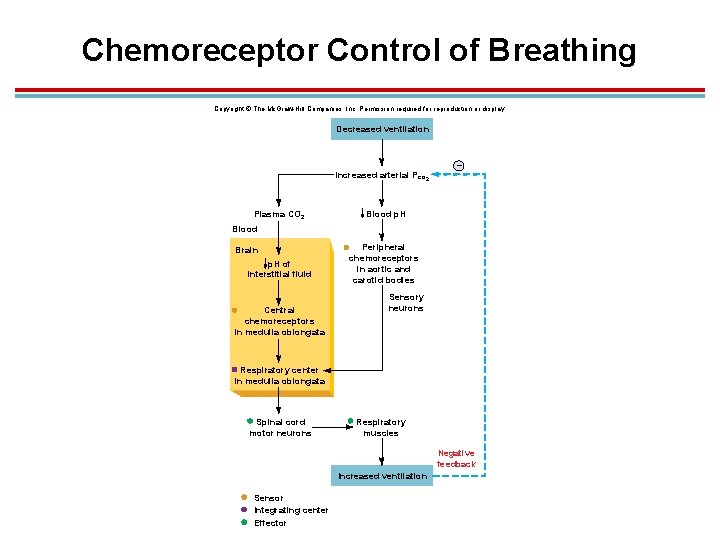
Chemoreceptor Control of Breathing Copyright © The Mc. Graw-Hill Companies, Inc. Permission required for reproduction or display. Decreased ventilation Increased arterial Pco 2 Plasma CO 2 – Blood p. H Blood Brain p. H of interstitial fluid Central chemoreceptors in medulla oblongata Peripheral chemoreceptors in aortic and carotid bodies Sensory neurons Respiratory center in medulla oblongata Spinal cord motor neurons Respiratory muscles Negative feedback Increased ventilation Sensor Integrating center Effector
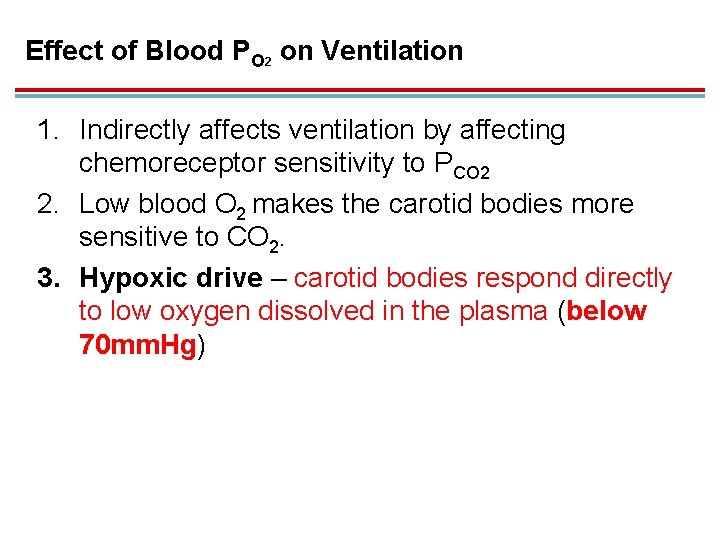
Effect of Blood PO 2 on Ventilation 1. Indirectly affects ventilation by affecting chemoreceptor sensitivity to PCO 2 2. Low blood O 2 makes the carotid bodies more sensitive to CO 2. 3. Hypoxic drive – carotid bodies respond directly to low oxygen dissolved in the plasma (below 70 mm. Hg)
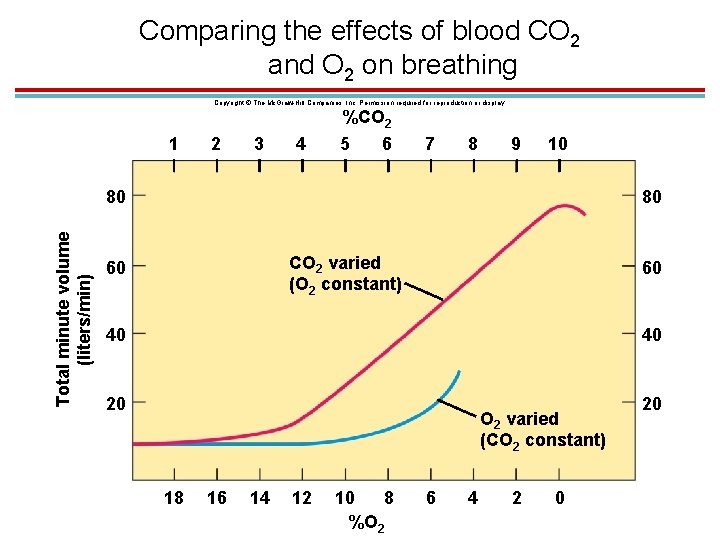
Comparing the effects of blood CO 2 and O 2 on breathing Copyright © The Mc. Graw-Hill Companies, Inc. Permission required for reproduction or display. 1 2 3 4 %CO 2 5 6 7 8 9 10 Total minute volume (liters/min) 80 80 CO 2 varied (O 2 constant) 60 60 40 40 20 20 O 2 varied (CO 2 constant) 18 16 14 12 10 8 %O 2 6 4 2 0
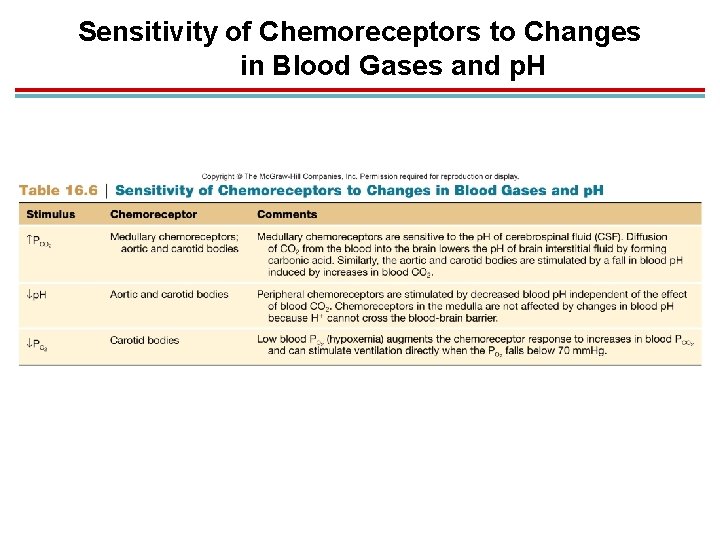
Sensitivity of Chemoreceptors to Changes in Blood Gases and p. H
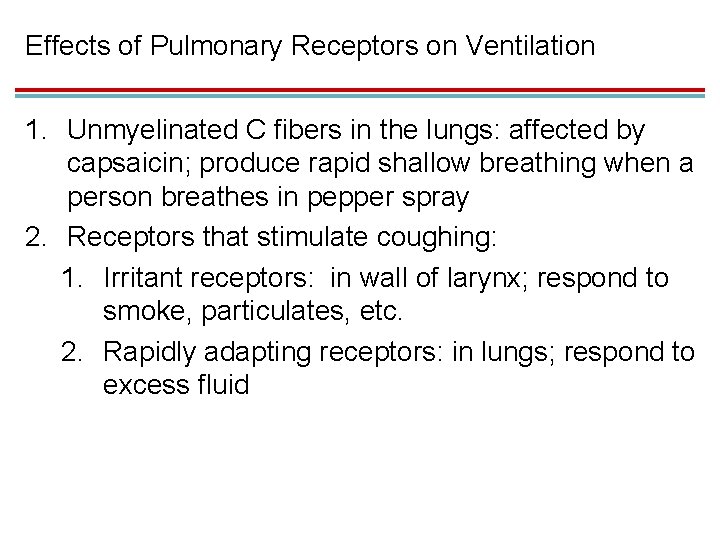
Effects of Pulmonary Receptors on Ventilation 1. Unmyelinated C fibers in the lungs: affected by capsaicin; produce rapid shallow breathing when a person breathes in pepper spray 2. Receptors that stimulate coughing: 1. Irritant receptors: in wall of larynx; respond to smoke, particulates, etc. 2. Rapidly adapting receptors: in lungs; respond to excess fluid
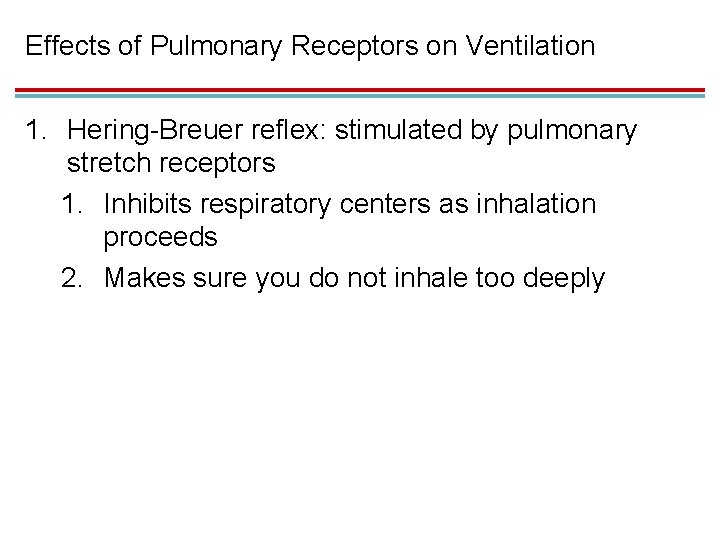
Effects of Pulmonary Receptors on Ventilation 1. Hering-Breuer reflex: stimulated by pulmonary stretch receptors 1. Inhibits respiratory centers as inhalation proceeds 2. Makes sure you do not inhale too deeply
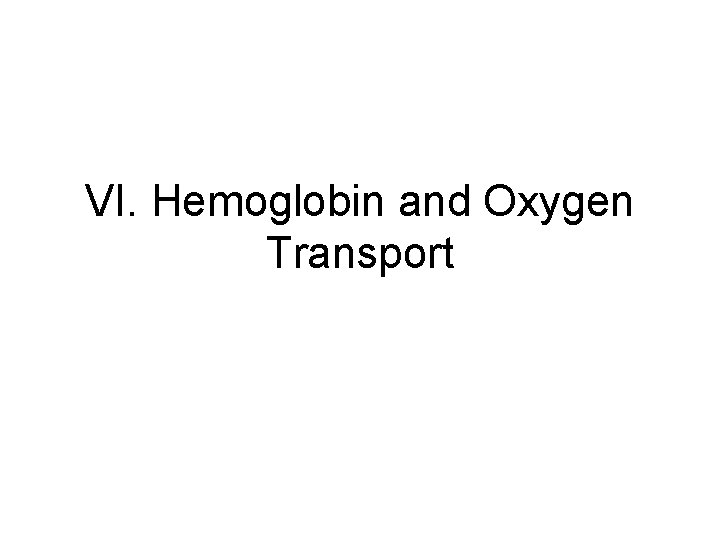
VI. Hemoglobin and Oxygen Transport
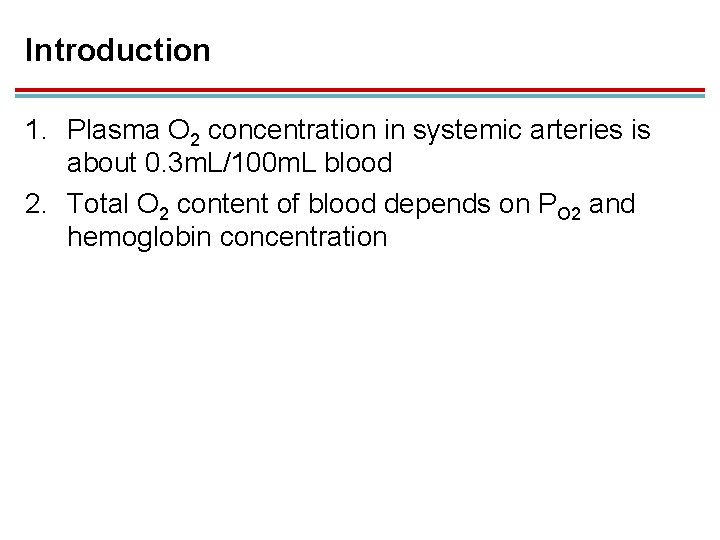
Introduction 1. Plasma O 2 concentration in systemic arteries is about 0. 3 m. L/100 m. L blood 2. Total O 2 content of blood depends on PO 2 and hemoglobin concentration
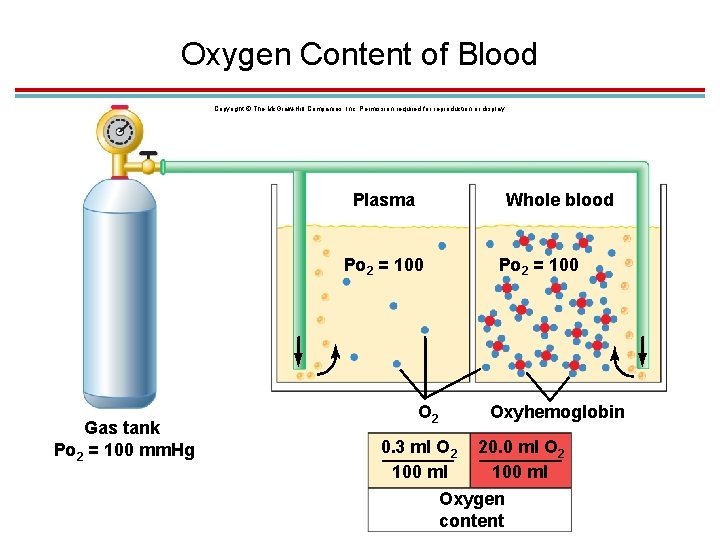
Oxygen Content of Blood Copyright © The Mc. Graw-Hill Companies, Inc. Permission required for reproduction or display. Plasma Whole blood Po 2 = 100 Gas tank Po 2 = 100 mm. Hg O 2 Po 2 = 100 Oxyhemoglobin 0. 3 ml O 2 20. 0 ml O 2 100 ml Oxygen content
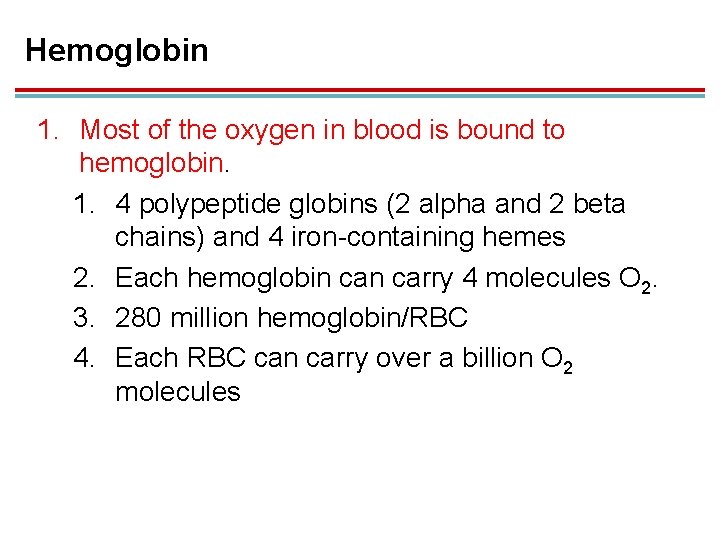
Hemoglobin 1. Most of the oxygen in blood is bound to hemoglobin. 1. 4 polypeptide globins (2 alpha and 2 beta chains) and 4 iron-containing hemes 2. Each hemoglobin carry 4 molecules O 2. 3. 280 million hemoglobin/RBC 4. Each RBC can carry over a billion O 2 molecules
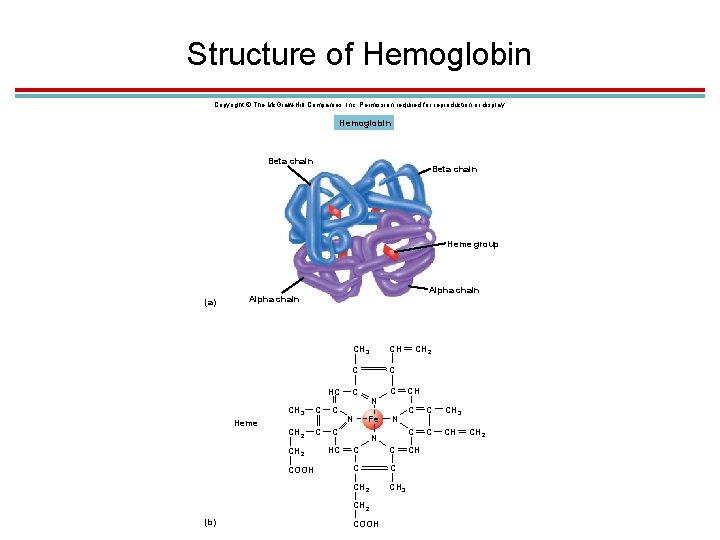
Structure of Hemoglobin Copyright © The Mc. Graw-Hill Companies, Inc. Permission required for reproduction or display. Hemoglobin Beta chain Heme group (a) Alpha chain HC Heme CH 3 C C CH 2 COOH HC CH 3 CH C C C N N Fe N N C C CH 2 CH 3 CH 2 (b) C COOH CH 2 CH C C CH 3 C C CH CH CH 2
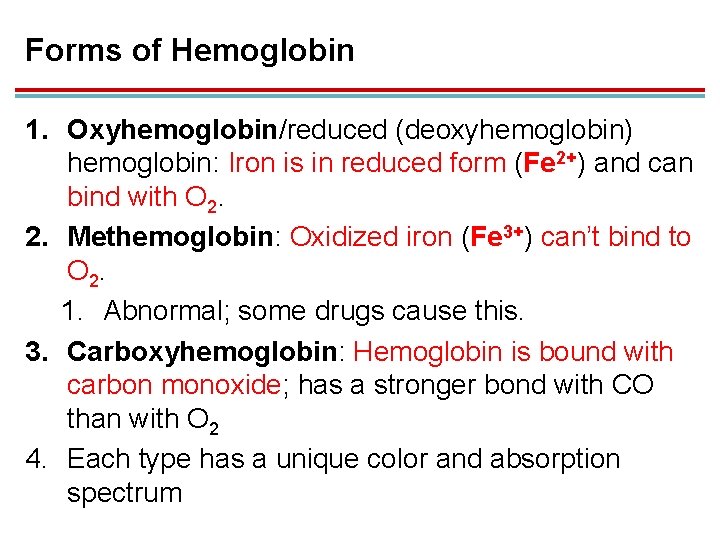
Forms of Hemoglobin 1. Oxyhemoglobin/reduced (deoxyhemoglobin) hemoglobin: Iron is in reduced form (Fe 2+) and can bind with O 2. 2. Methemoglobin: Oxidized iron (Fe 3+) can’t bind to O 2. 1. Abnormal; some drugs cause this. 3. Carboxyhemoglobin: Hemoglobin is bound with carbon monoxide; has a stronger bond with CO than with O 2 4. Each type has a unique color and absorption spectrum
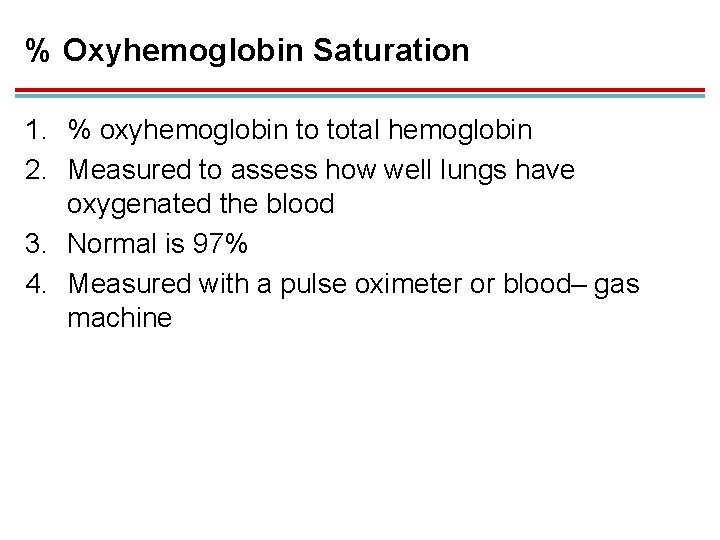
% Oxyhemoglobin Saturation 1. % oxyhemoglobin to total hemoglobin 2. Measured to assess how well lungs have oxygenated the blood 3. Normal is 97% 4. Measured with a pulse oximeter or blood– gas machine
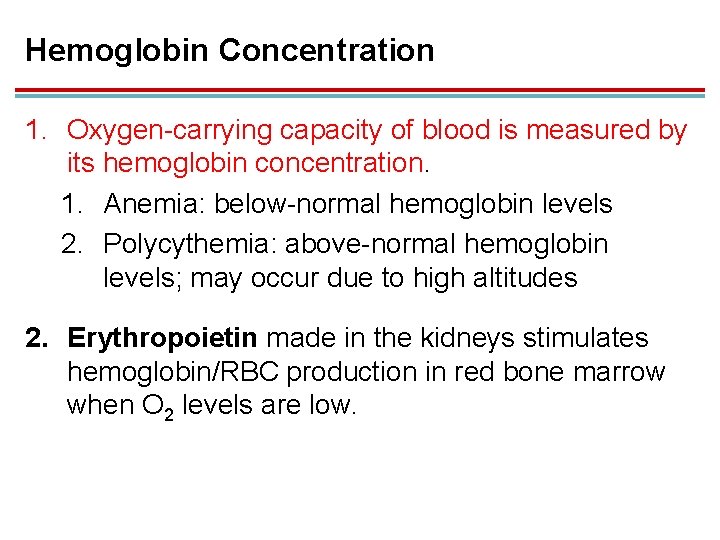
Hemoglobin Concentration 1. Oxygen-carrying capacity of blood is measured by its hemoglobin concentration. 1. Anemia: below-normal hemoglobin levels 2. Polycythemia: above-normal hemoglobin levels; may occur due to high altitudes 2. Erythropoietin made in the kidneys stimulates hemoglobin/RBC production in red bone marrow when O 2 levels are low.
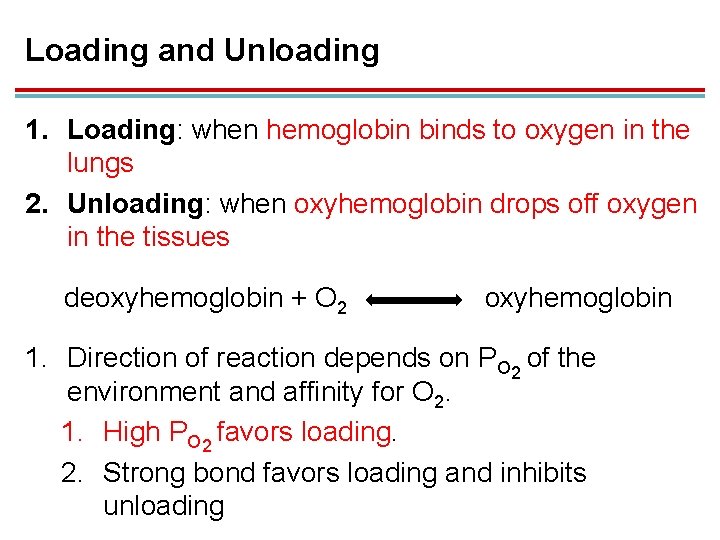
Loading and Unloading 1. Loading: when hemoglobin binds to oxygen in the lungs 2. Unloading: when oxyhemoglobin drops off oxygen in the tissues deoxyhemoglobin + O 2 oxyhemoglobin 1. Direction of reaction depends on PO 2 of the environment and affinity for O 2. 1. High PO 2 favors loading. 2. Strong bond favors loading and inhibits unloading
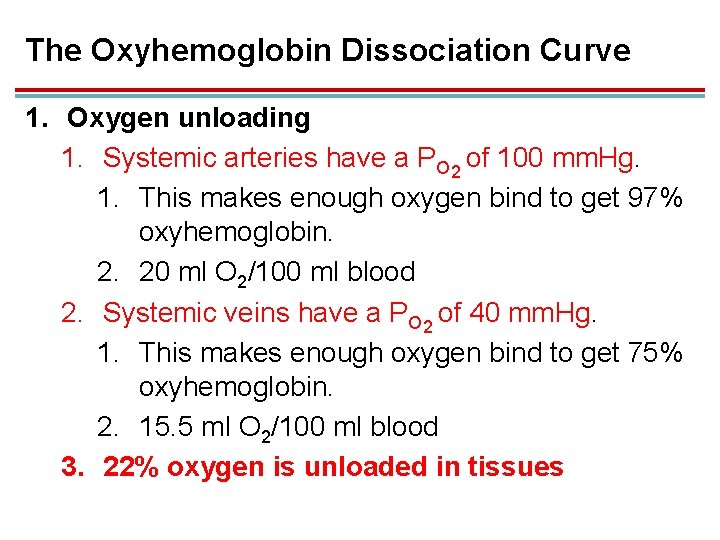
The Oxyhemoglobin Dissociation Curve 1. Oxygen unloading 1. Systemic arteries have a PO 2 of 100 mm. Hg. 1. This makes enough oxygen bind to get 97% oxyhemoglobin. 2. 20 ml O 2/100 ml blood 2. Systemic veins have a PO 2 of 40 mm. Hg. 1. This makes enough oxygen bind to get 75% oxyhemoglobin. 2. 15. 5 ml O 2/100 ml blood 3. 22% oxygen is unloaded in tissues
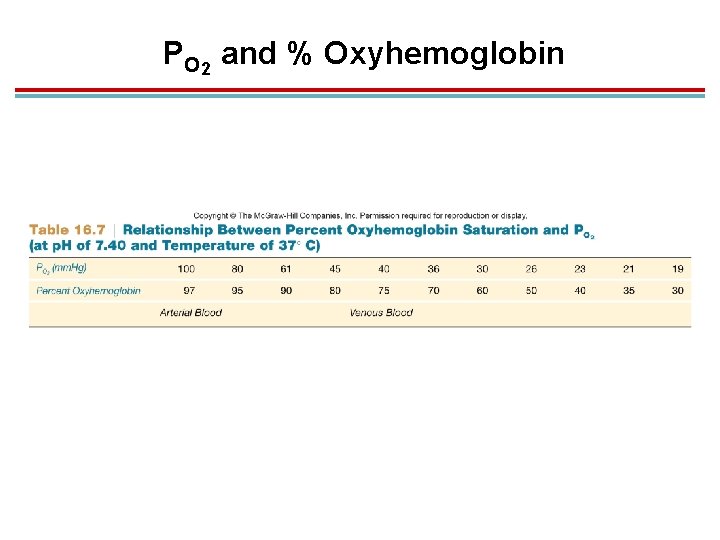
PO 2 and % Oxyhemoglobin
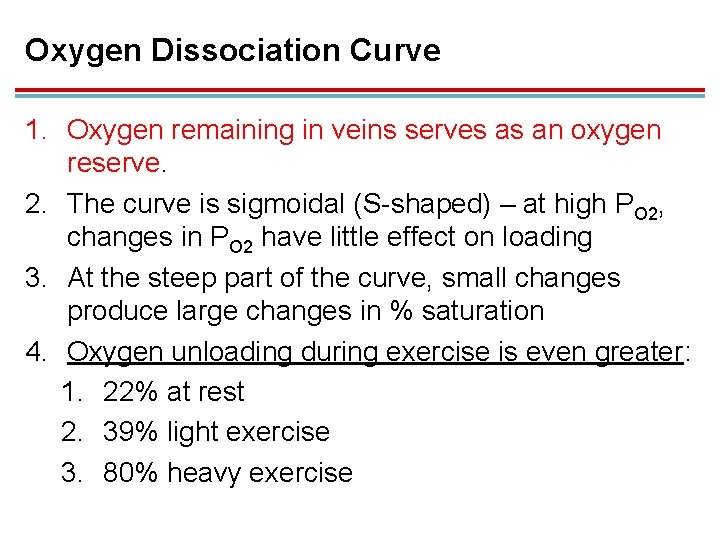
Oxygen Dissociation Curve 1. Oxygen remaining in veins serves as an oxygen reserve. 2. The curve is sigmoidal (S-shaped) – at high PO 2, changes in PO 2 have little effect on loading 3. At the steep part of the curve, small changes produce large changes in % saturation 4. Oxygen unloading during exercise is even greater: 1. 22% at rest 2. 39% light exercise 3. 80% heavy exercise
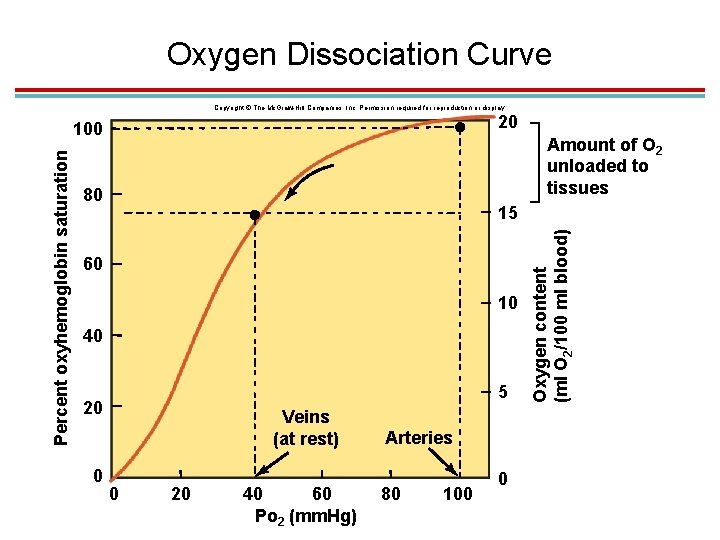
Oxygen Dissociation Curve Copyright © The Mc. Graw-Hill Companies, Inc. Permission required for reproduction or display. 20 Amount of O 2 unloaded to tissues 80 15 60 10 40 5 20 0 Veins (at rest) 0 20 40 60 Po 2 (mm. Hg) Arteries 80 100 0 Oxygen content (ml O 2/100 ml blood) Percent oxyhemoglobin saturation 100
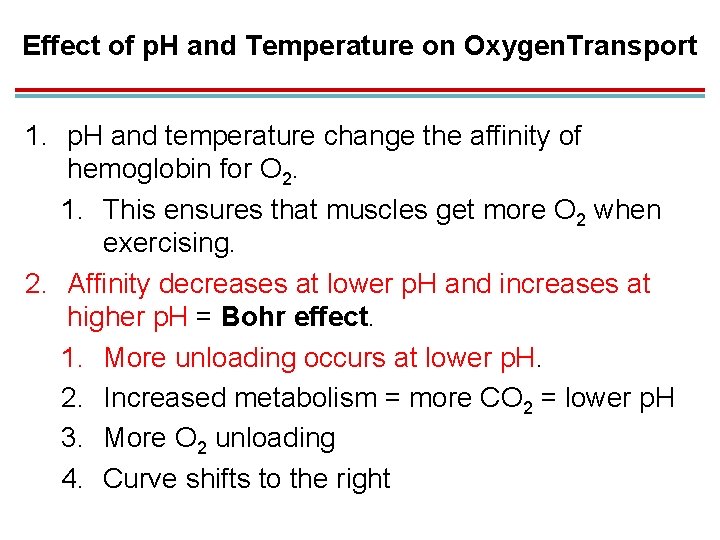
Effect of p. H and Temperature on Oxygen. Transport 1. p. H and temperature change the affinity of hemoglobin for O 2. 1. This ensures that muscles get more O 2 when exercising. 2. Affinity decreases at lower p. H and increases at higher p. H = Bohr effect. 1. More unloading occurs at lower p. H. 2. Increased metabolism = more CO 2 = lower p. H 3. More O 2 unloading 4. Curve shifts to the right
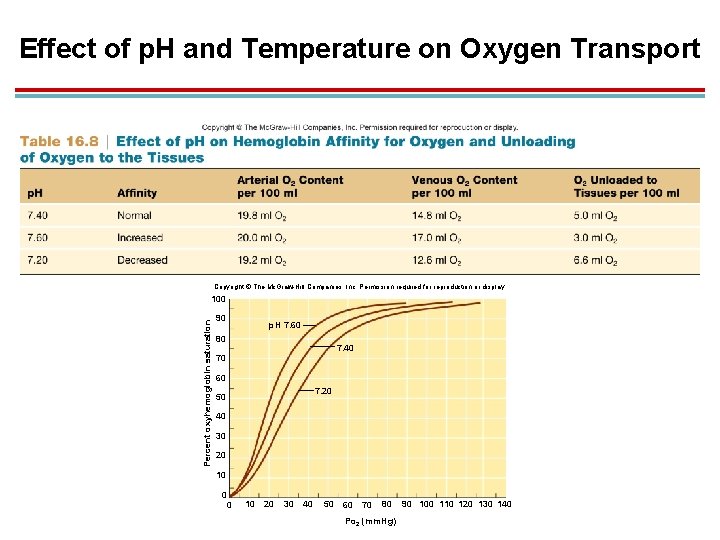
Effect of p. H and Temperature on Oxygen Transport Copyright © The Mc. Graw-Hill Companies, Inc. Permission required for reproduction or display. Percent oxyhemoglobin saturation 100 90 p. H 7. 60 80 7. 40 70 60 7. 20 50 40 30 20 10 0 0 10 20 30 40 50 60 70 80 Po 2 (mm. Hg) 90 100 110 120 130 140
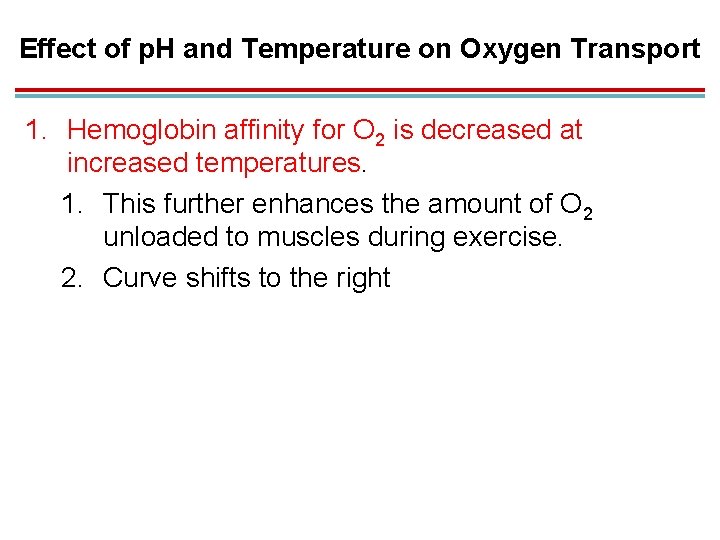
Effect of p. H and Temperature on Oxygen Transport 1. Hemoglobin affinity for O 2 is decreased at increased temperatures. 1. This further enhances the amount of O 2 unloaded to muscles during exercise. 2. Curve shifts to the right
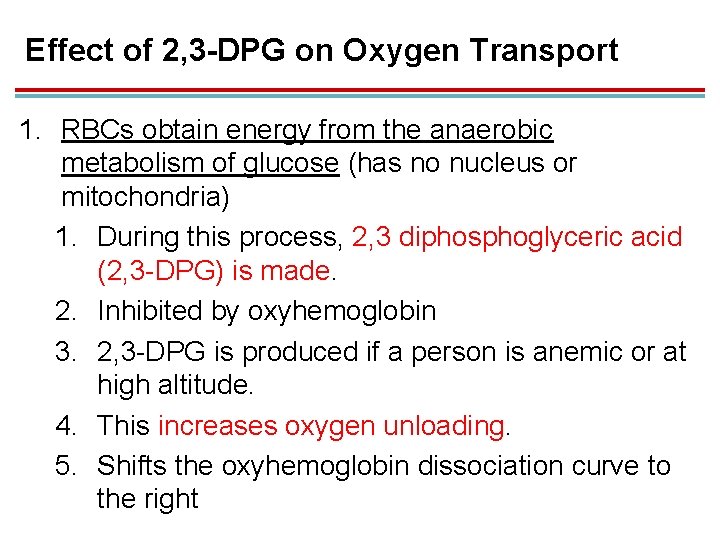
Effect of 2, 3 -DPG on Oxygen Transport 1. RBCs obtain energy from the anaerobic metabolism of glucose (has no nucleus or mitochondria) 1. During this process, 2, 3 diphosphoglyceric acid (2, 3 -DPG) is made. 2. Inhibited by oxyhemoglobin 3. 2, 3 -DPG is produced if a person is anemic or at high altitude. 4. This increases oxygen unloading. 5. Shifts the oxyhemoglobin dissociation curve to the right
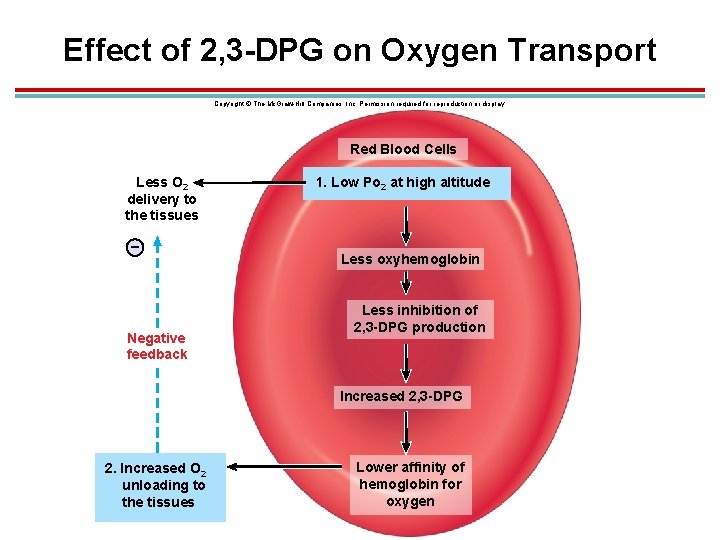
Effect of 2, 3 -DPG on Oxygen Transport Copyright © The Mc. Graw-Hill Companies, Inc. Permission required for reproduction or display. Red Blood Cells Less O 2 delivery to the tissues – Negative feedback 1. Low Po 2 at high altitude Less oxyhemoglobin Less inhibition of 2, 3 -DPG production Increased 2, 3 -DPG 2. Increased O 2 unloading to the tissues Lower affinity of hemoglobin for oxygen
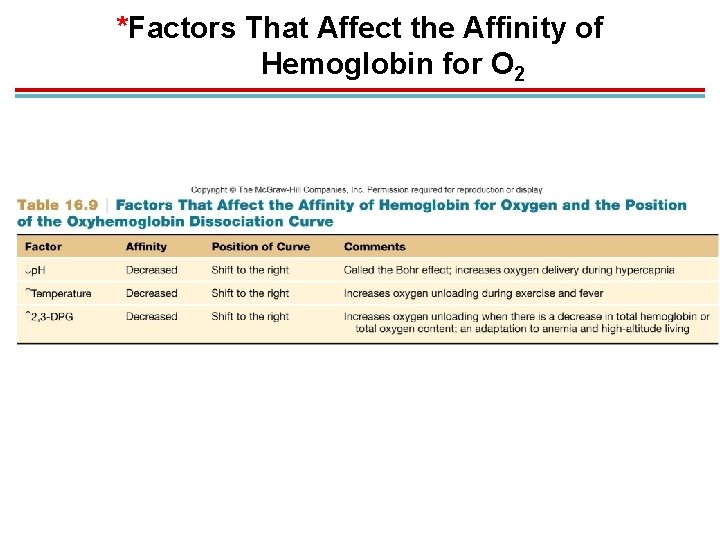
*Factors That Affect the Affinity of Hemoglobin for O 2
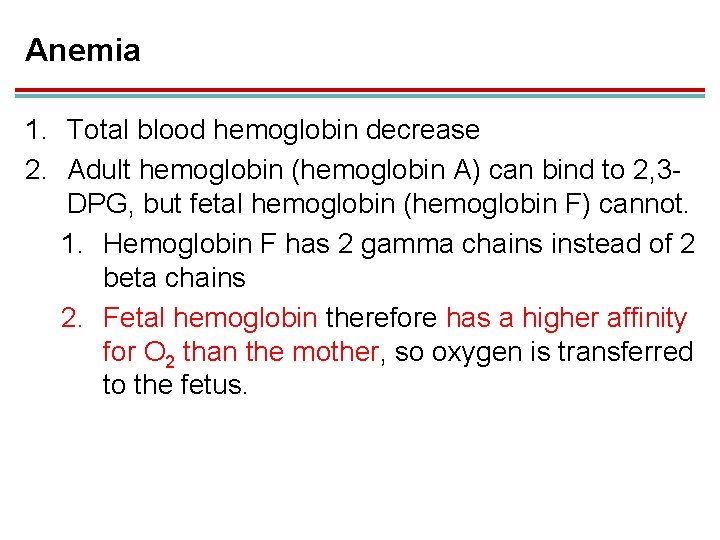
Anemia 1. Total blood hemoglobin decrease 2. Adult hemoglobin (hemoglobin A) can bind to 2, 3 DPG, but fetal hemoglobin (hemoglobin F) cannot. 1. Hemoglobin F has 2 gamma chains instead of 2 beta chains 2. Fetal hemoglobin therefore has a higher affinity for O 2 than the mother, so oxygen is transferred to the fetus.
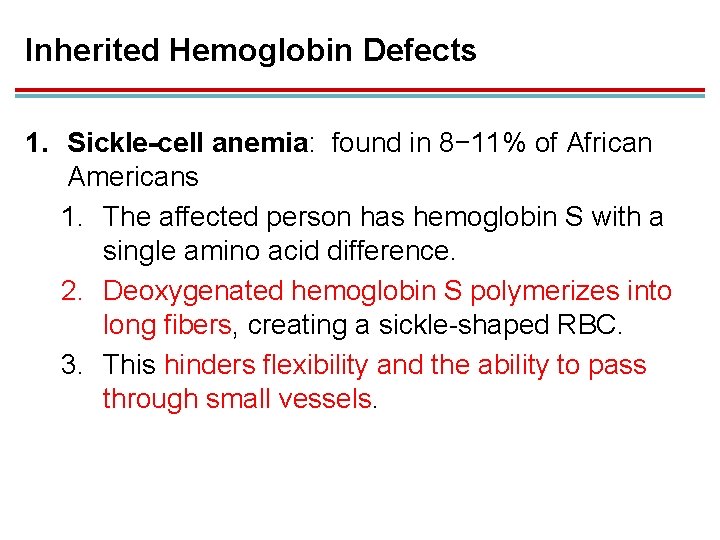
Inherited Hemoglobin Defects 1. Sickle-cell anemia: found in 8− 11% of African Americans 1. The affected person has hemoglobin S with a single amino acid difference. 2. Deoxygenated hemoglobin S polymerizes into long fibers, creating a sickle-shaped RBC. 3. This hinders flexibility and the ability to pass through small vessels.
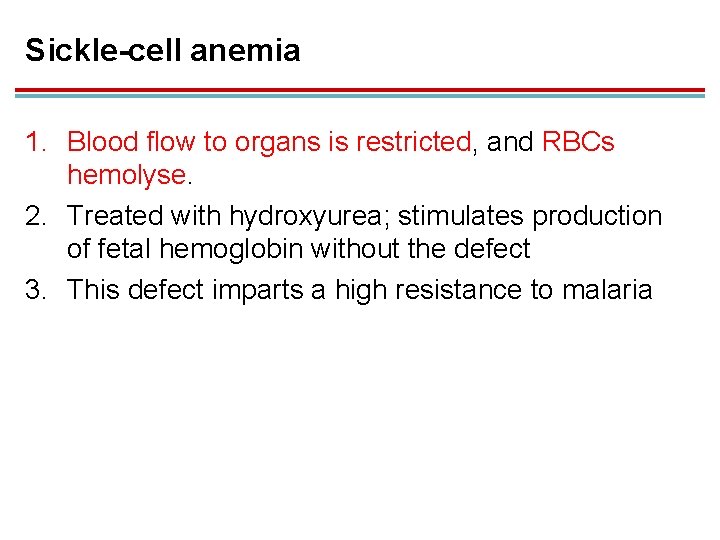
Sickle-cell anemia 1. Blood flow to organs is restricted, and RBCs hemolyse. 2. Treated with hydroxyurea; stimulates production of fetal hemoglobin without the defect 3. This defect imparts a high resistance to malaria
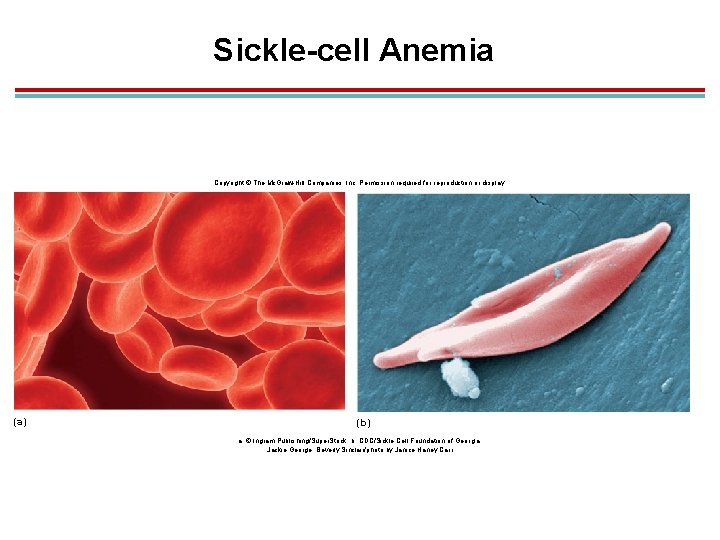
Sickle-cell Anemia Copyright © The Mc. Graw-Hill Companies, Inc. Permission required for reproduction or display. (a) (b) a: © Ingram Publishing/Super. Stock; b: CDC/Sickle Cell Foundation of Georgia: Jackie George, Beverly Sinclair/photo by Janice Haney Carr
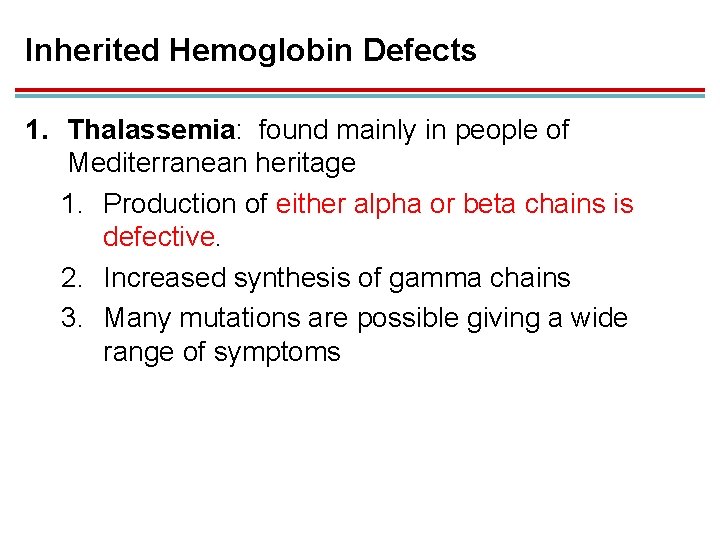
Inherited Hemoglobin Defects 1. Thalassemia: found mainly in people of Mediterranean heritage 1. Production of either alpha or beta chains is defective. 2. Increased synthesis of gamma chains 3. Many mutations are possible giving a wide range of symptoms
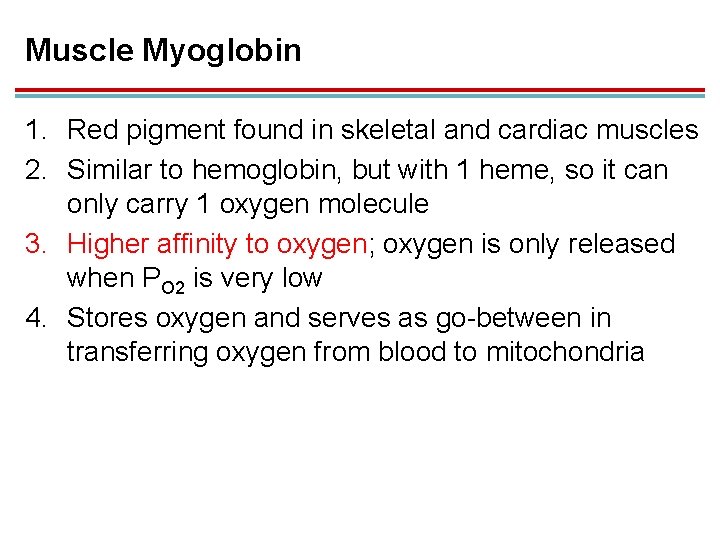
Muscle Myoglobin 1. Red pigment found in skeletal and cardiac muscles 2. Similar to hemoglobin, but with 1 heme, so it can only carry 1 oxygen molecule 3. Higher affinity to oxygen; oxygen is only released when PO 2 is very low 4. Stores oxygen and serves as go-between in transferring oxygen from blood to mitochondria
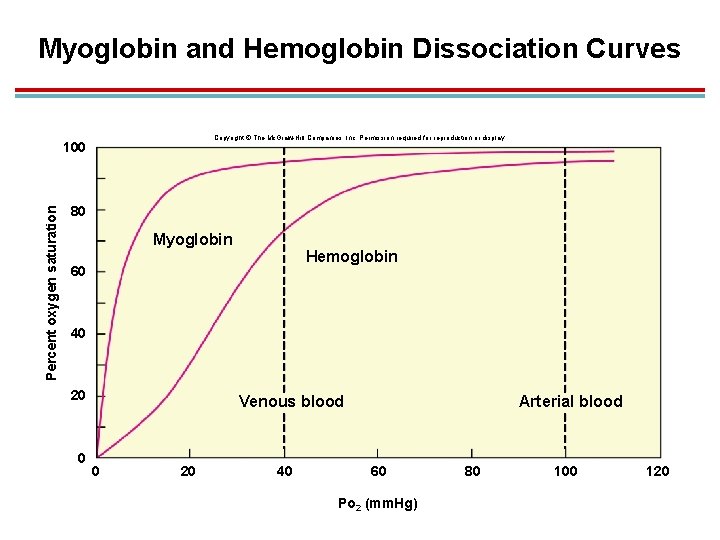
Myoglobin and Hemoglobin Dissociation Curves Copyright © The Mc. Graw-Hill Companies, Inc. Permission required for reproduction or display. Percent oxygen saturation 100 80 Myoglobin Hemoglobin 60 40 20 0 Venous blood 0 20 40 Arterial blood 60 Po 2 (mm. Hg) 80 100 120
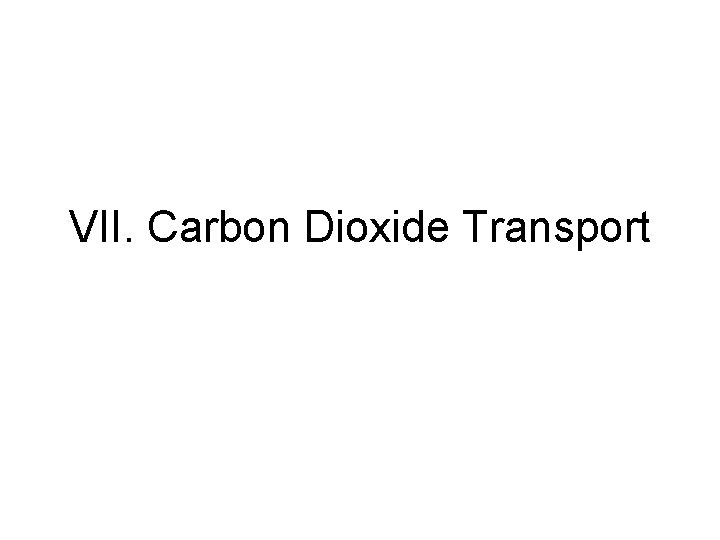
VII. Carbon Dioxide Transport
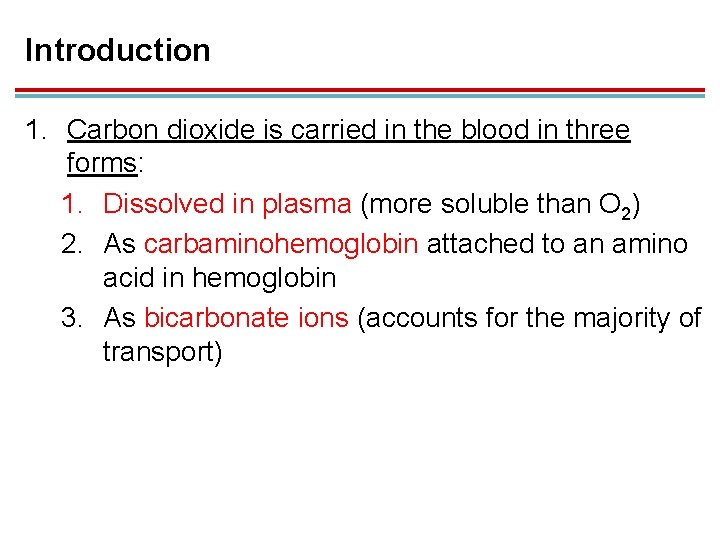
Introduction 1. Carbon dioxide is carried in the blood in three forms: 1. Dissolved in plasma (more soluble than O 2) 2. As carbaminohemoglobin attached to an amino acid in hemoglobin 3. As bicarbonate ions (accounts for the majority of transport)
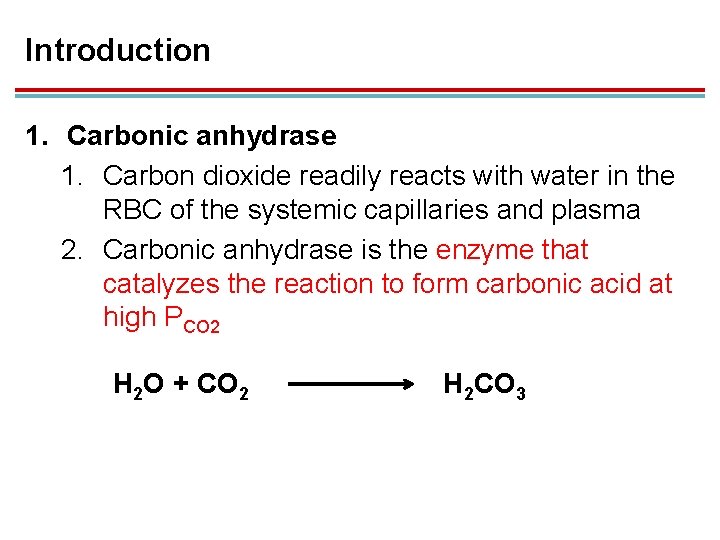
Introduction 1. Carbonic anhydrase 1. Carbon dioxide readily reacts with water in the RBC of the systemic capillaries and plasma 2. Carbonic anhydrase is the enzyme that catalyzes the reaction to form carbonic acid at high PCO 2 H 2 O + CO 2 H 2 CO 3
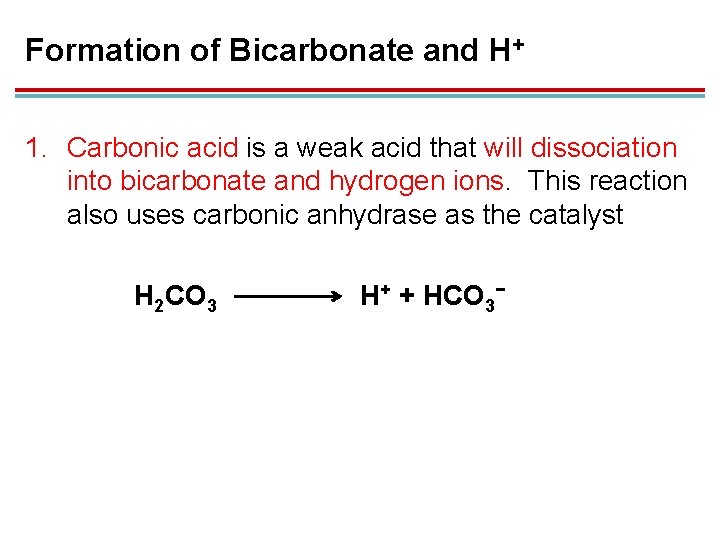
Formation of Bicarbonate and H+ 1. Carbonic acid is a weak acid that will dissociation into bicarbonate and hydrogen ions. This reaction also uses carbonic anhydrase as the catalyst H 2 CO 3 H+ + HCO 3−
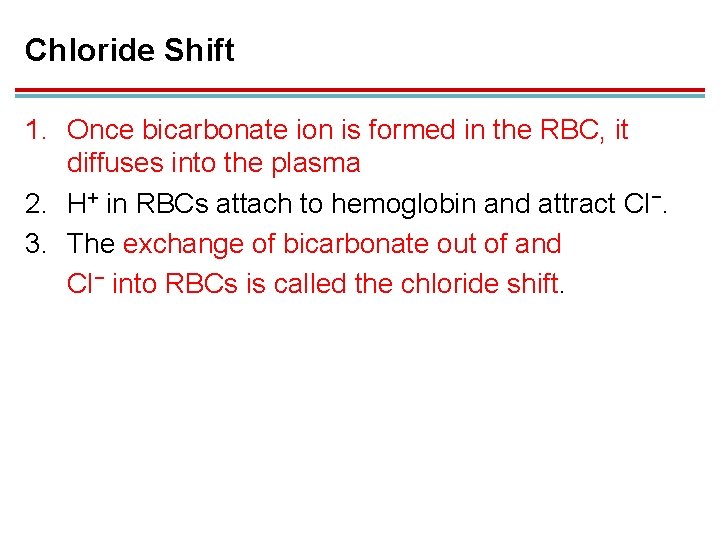
Chloride Shift 1. Once bicarbonate ion is formed in the RBC, it diffuses into the plasma 2. H+ in RBCs attach to hemoglobin and attract Cl−. 3. The exchange of bicarbonate out of and Cl− into RBCs is called the chloride shift.
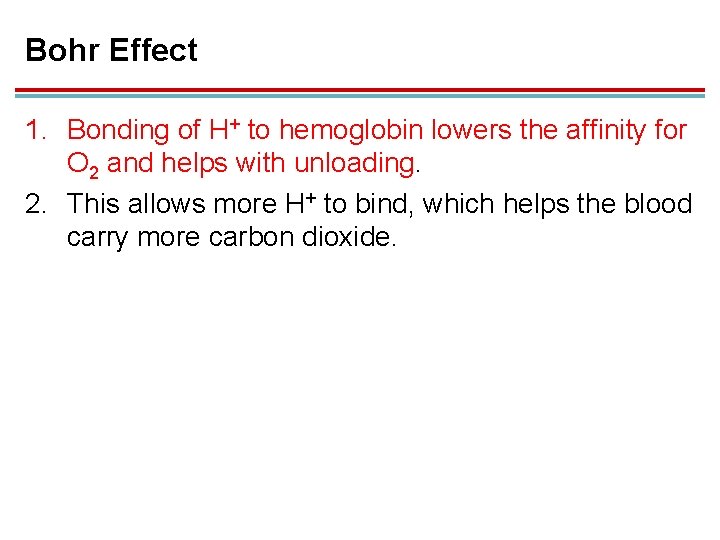
Bohr Effect 1. Bonding of H+ to hemoglobin lowers the affinity for O 2 and helps with unloading. 2. This allows more H+ to bind, which helps the blood carry more carbon dioxide.
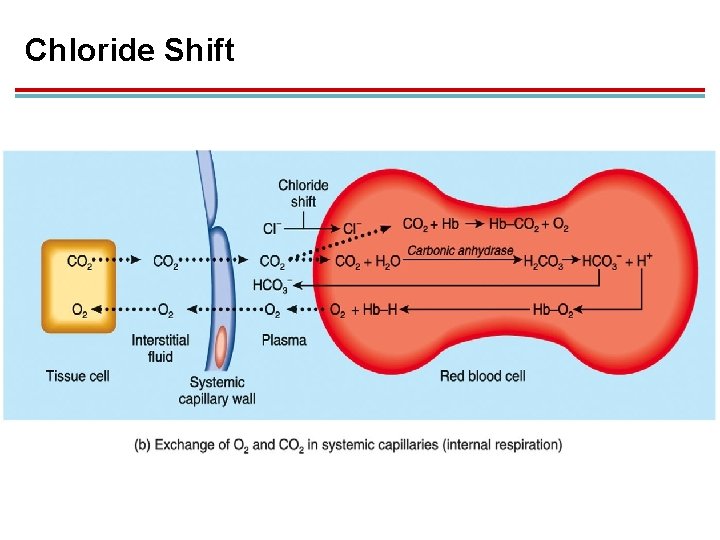
Chloride Shift
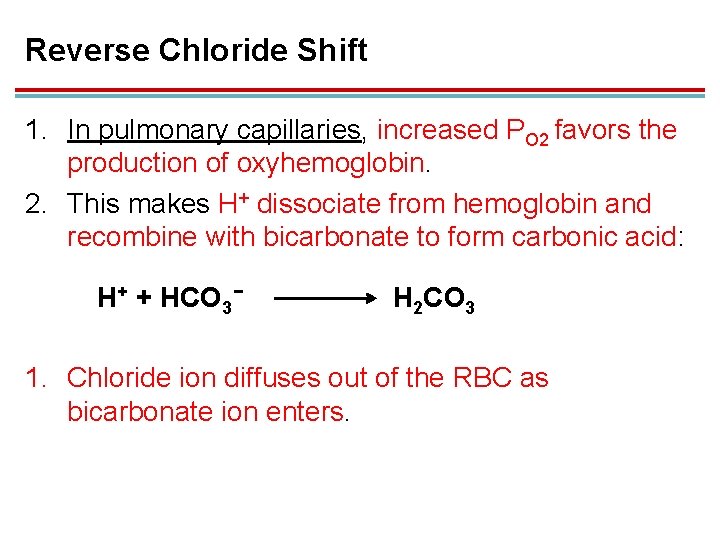
Reverse Chloride Shift 1. In pulmonary capillaries, increased PO 2 favors the production of oxyhemoglobin. 2. This makes H+ dissociate from hemoglobin and recombine with bicarbonate to form carbonic acid: H+ + HCO 3− H 2 CO 3 1. Chloride ion diffuses out of the RBC as bicarbonate ion enters.
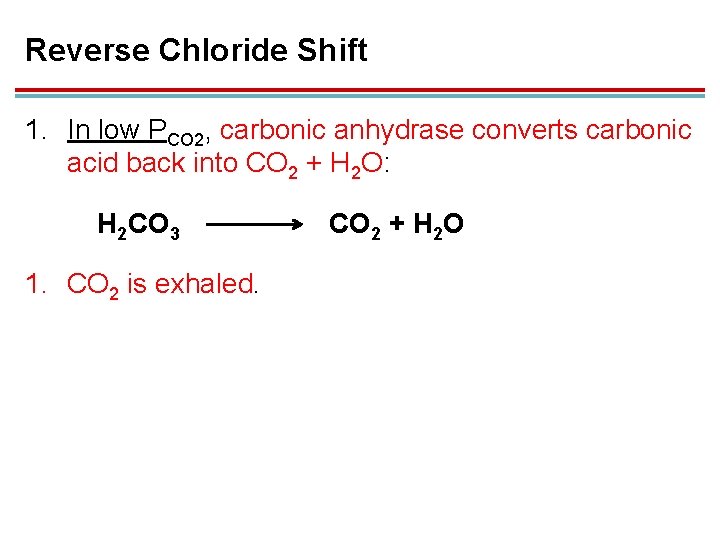
Reverse Chloride Shift 1. In low PCO 2, carbonic anhydrase converts carbonic acid back into CO 2 + H 2 O: H 2 CO 3 1. CO 2 is exhaled. CO 2 + H 2 O
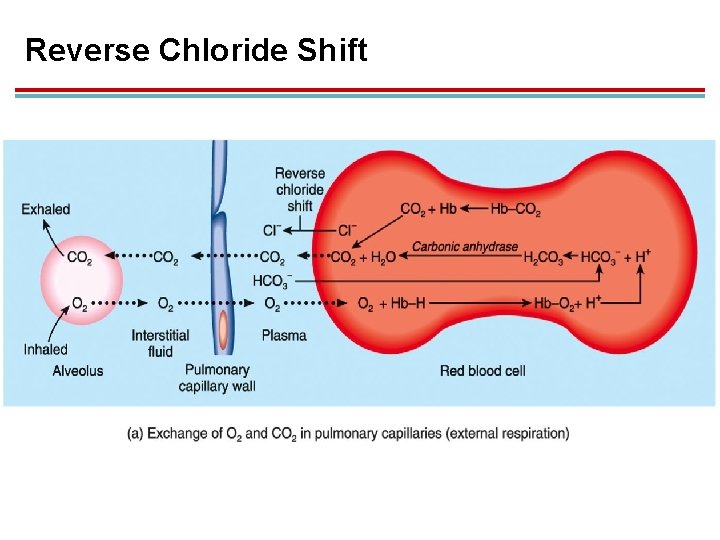
Reverse Chloride Shift
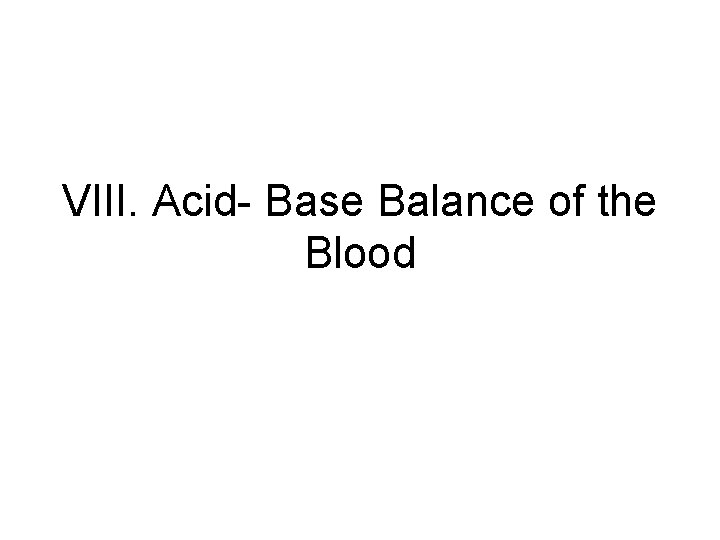
VIII. Acid- Base Balance of the Blood
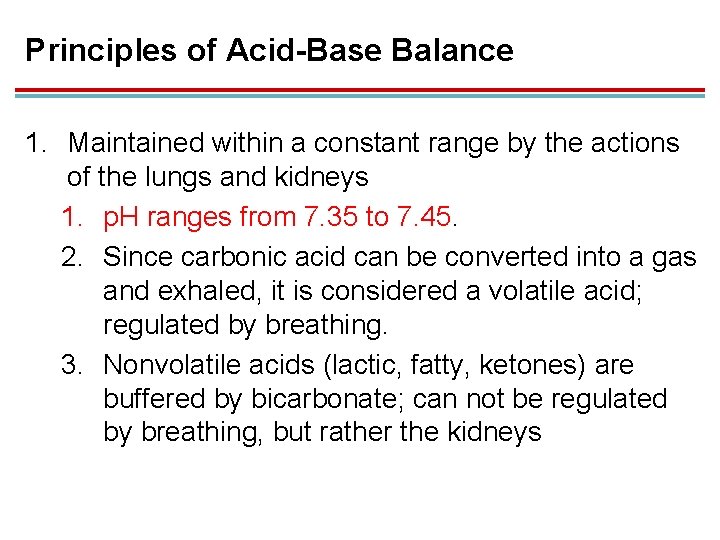
Principles of Acid-Base Balance 1. Maintained within a constant range by the actions of the lungs and kidneys 1. p. H ranges from 7. 35 to 7. 45. 2. Since carbonic acid can be converted into a gas and exhaled, it is considered a volatile acid; regulated by breathing. 3. Nonvolatile acids (lactic, fatty, ketones) are buffered by bicarbonate; can not be regulated by breathing, but rather the kidneys
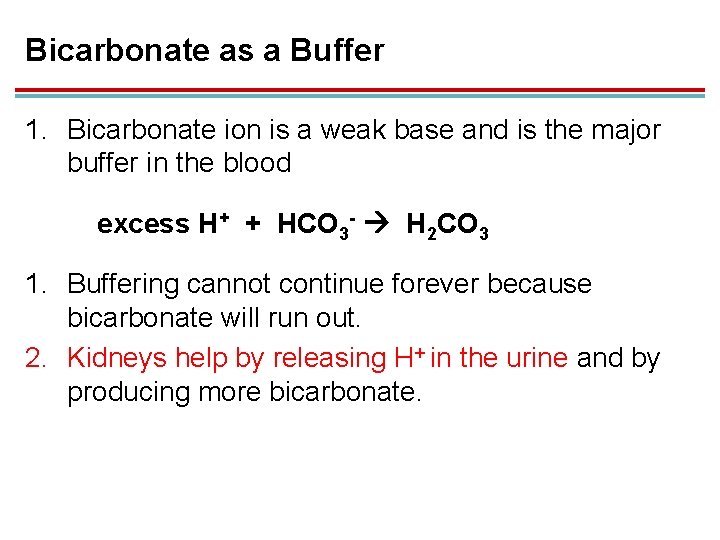
Bicarbonate as a Buffer 1. Bicarbonate ion is a weak base and is the major buffer in the blood excess H+ + HCO 3 - H 2 CO 3 1. Buffering cannot continue forever because bicarbonate will run out. 2. Kidneys help by releasing H+ in the urine and by producing more bicarbonate.
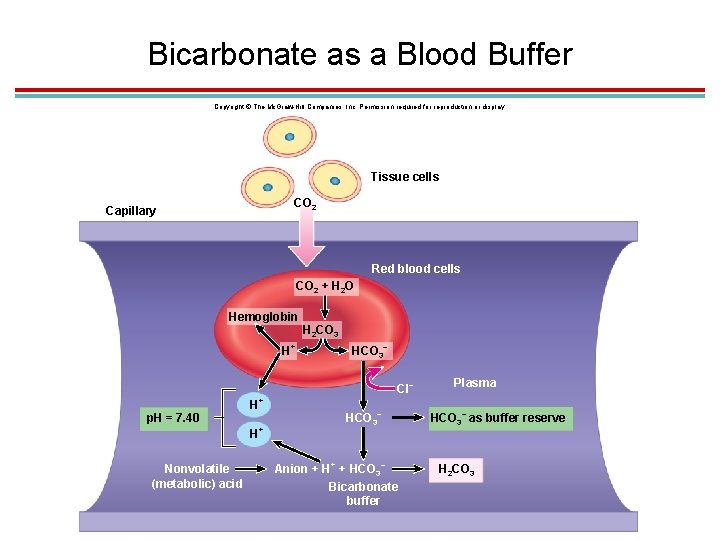
Bicarbonate as a Blood Buffer Copyright © The Mc. Graw-Hill Companies, Inc. Permission required for reproduction or display. Tissue cells CO 2 Capillary Red blood cells CO 2 + H 2 O Hemoglobin H 2 CO 3 + – H HCO 3 Cl + p. H = 7. 40 H – HCO 3 + – Plasma – HCO 3 as buffer reserve H Nonvolatile (metabolic) acid + – Anion + HCO 3 Bicarbonate buffer H 2 CO 3
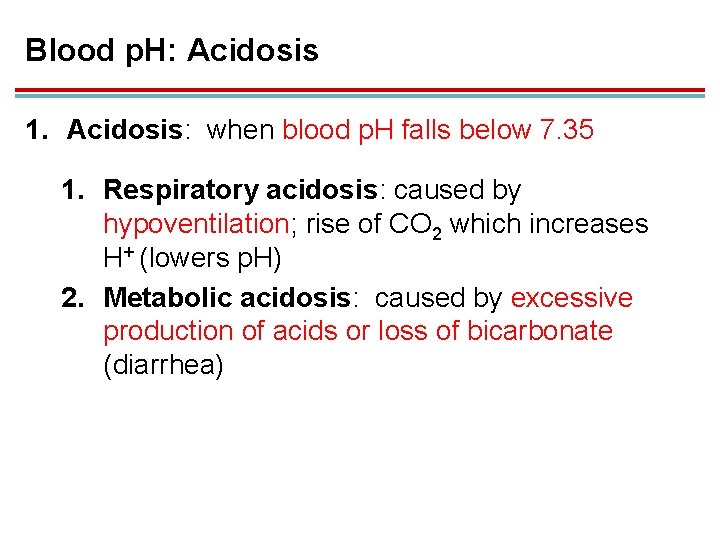
Blood p. H: Acidosis 1. Acidosis: when blood p. H falls below 7. 35 1. Respiratory acidosis: caused by hypoventilation; rise of CO 2 which increases H+ (lowers p. H) 2. Metabolic acidosis: caused by excessive production of acids or loss of bicarbonate (diarrhea)
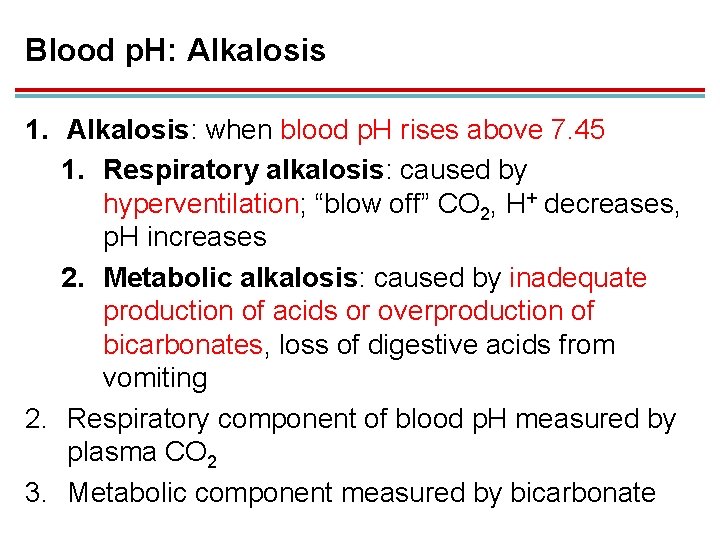
Blood p. H: Alkalosis 1. Alkalosis: when blood p. H rises above 7. 45 1. Respiratory alkalosis: caused by hyperventilation; “blow off” CO 2, H+ decreases, p. H increases 2. Metabolic alkalosis: caused by inadequate production of acids or overproduction of bicarbonates, loss of digestive acids from vomiting 2. Respiratory component of blood p. H measured by plasma CO 2 3. Metabolic component measured by bicarbonate
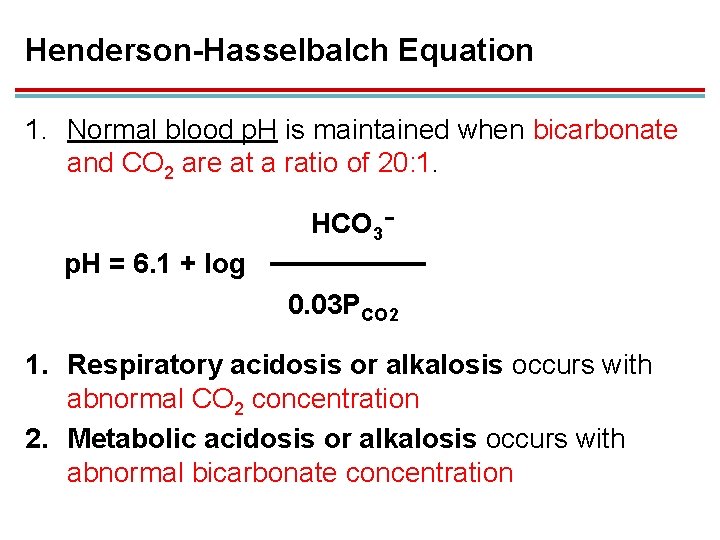
Henderson-Hasselbalch Equation 1. Normal blood p. H is maintained when bicarbonate and CO 2 are at a ratio of 20: 1. HCO 3− p. H = 6. 1 + log 0. 03 PCO 2 1. Respiratory acidosis or alkalosis occurs with abnormal CO 2 concentration 2. Metabolic acidosis or alkalosis occurs with abnormal bicarbonate concentration
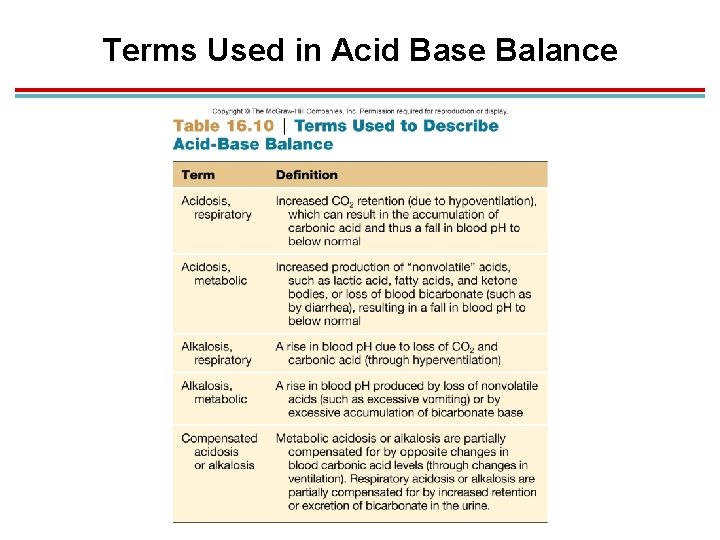
Terms Used in Acid Base Balance
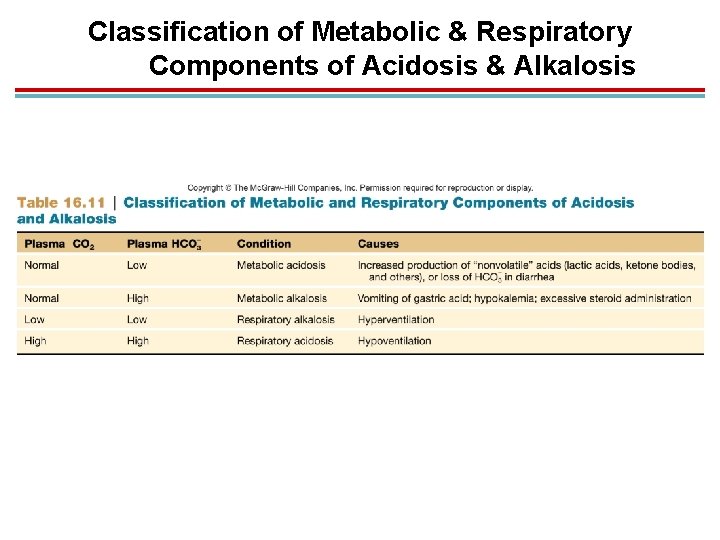
Classification of Metabolic & Respiratory Components of Acidosis & Alkalosis
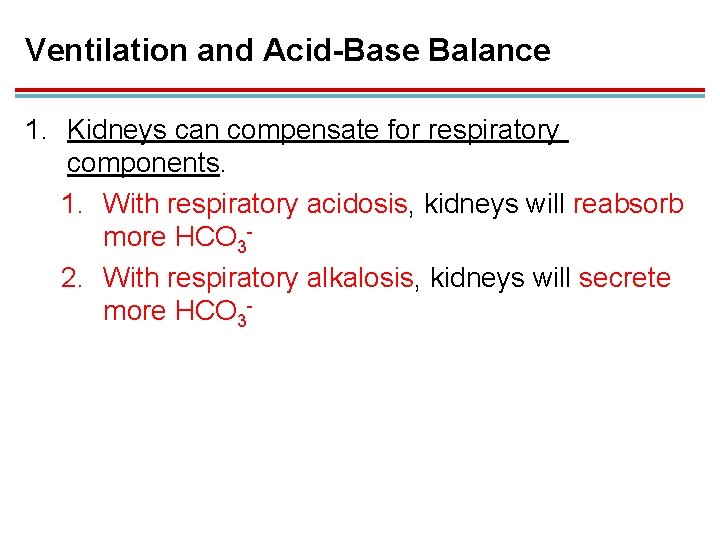
Ventilation and Acid-Base Balance 1. Kidneys can compensate for respiratory components. 1. With respiratory acidosis, kidneys will reabsorb more HCO 32. With respiratory alkalosis, kidneys will secrete more HCO 3 -
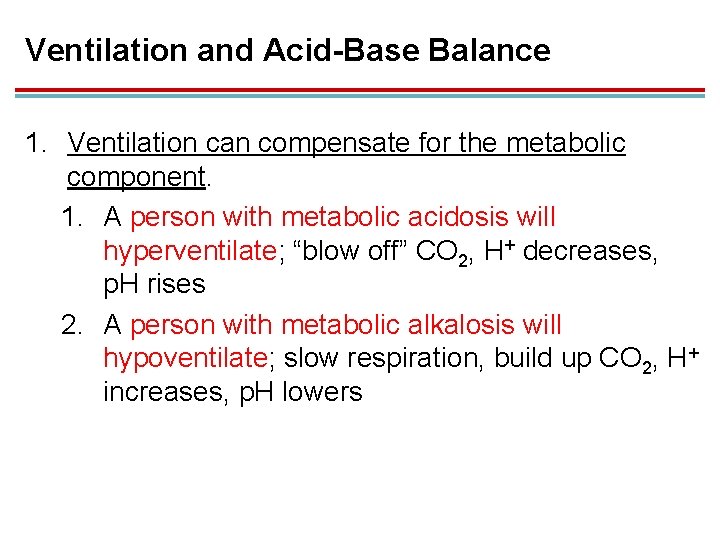
Ventilation and Acid-Base Balance 1. Ventilation can compensate for the metabolic component. 1. A person with metabolic acidosis will hyperventilate; “blow off” CO 2, H+ decreases, p. H rises 2. A person with metabolic alkalosis will hypoventilate; slow respiration, build up CO 2, H+ increases, p. H lowers
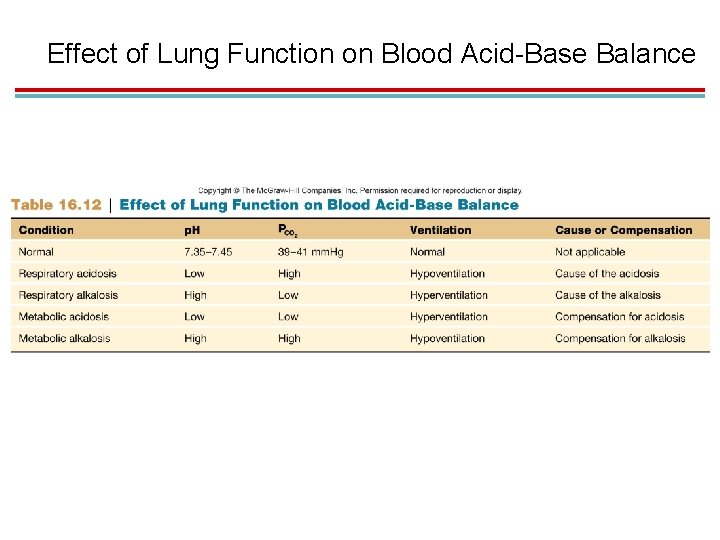
Effect of Lung Function on Blood Acid-Base Balance
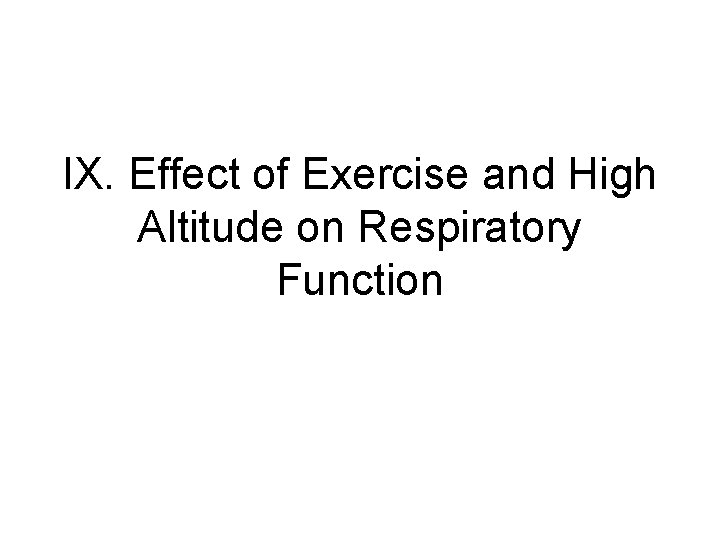
IX. Effect of Exercise and High Altitude on Respiratory Function
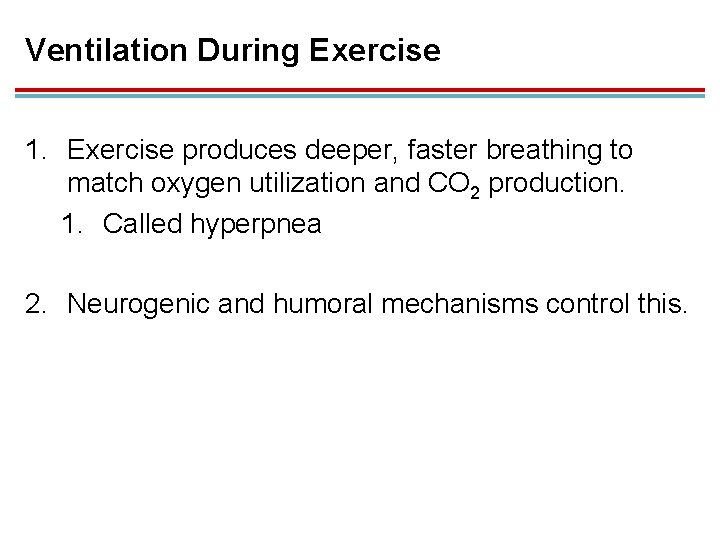
Ventilation During Exercise 1. Exercise produces deeper, faster breathing to match oxygen utilization and CO 2 production. 1. Called hyperpnea 2. Neurogenic and humoral mechanisms control this.
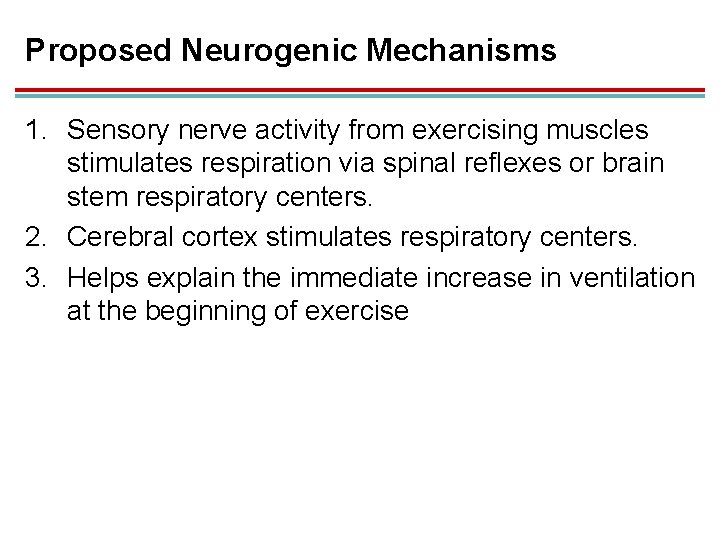
Proposed Neurogenic Mechanisms 1. Sensory nerve activity from exercising muscles stimulates respiration via spinal reflexes or brain stem respiratory centers. 2. Cerebral cortex stimulates respiratory centers. 3. Helps explain the immediate increase in ventilation at the beginning of exercise
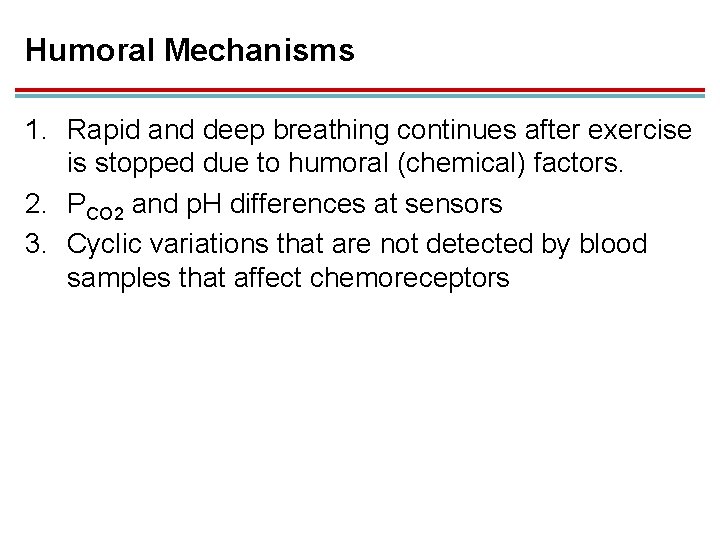
Humoral Mechanisms 1. Rapid and deep breathing continues after exercise is stopped due to humoral (chemical) factors. 2. PCO 2 and p. H differences at sensors 3. Cyclic variations that are not detected by blood samples that affect chemoreceptors
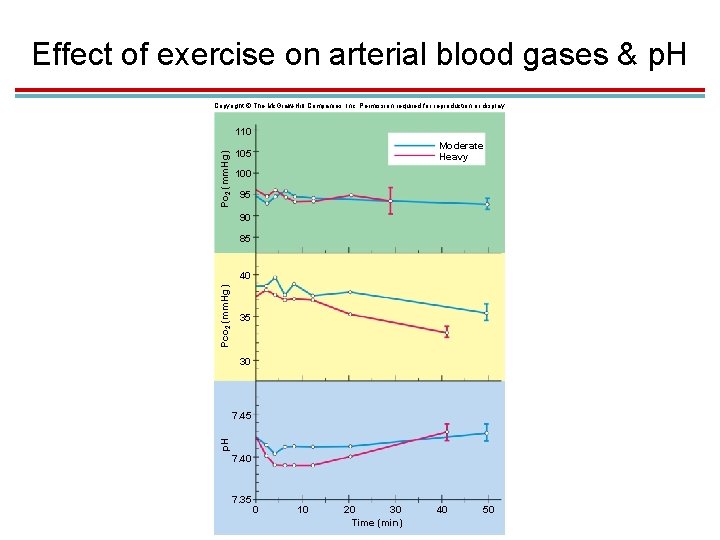
Effect of exercise on arterial blood gases & p. H Copyright © The Mc. Graw-Hill Companies, Inc. Permission required for reproduction or display. Po 2 (mm. Hg) 110 Moderate Heavy 105 100 95 90 85 Pco 2 (mm. Hg) 40 35 30 p. H 7. 45 7. 40 7. 35 0 10 20 30 Time (min) 40 50
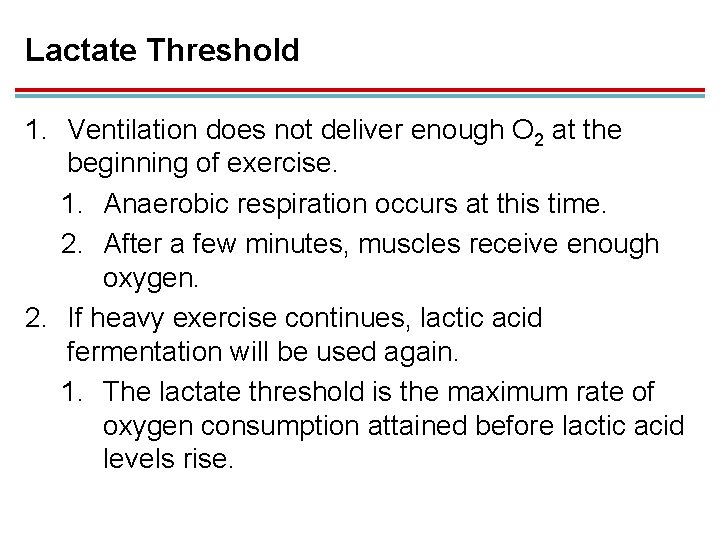
Lactate Threshold 1. Ventilation does not deliver enough O 2 at the beginning of exercise. 1. Anaerobic respiration occurs at this time. 2. After a few minutes, muscles receive enough oxygen. 2. If heavy exercise continues, lactic acid fermentation will be used again. 1. The lactate threshold is the maximum rate of oxygen consumption attained before lactic acid levels rise.
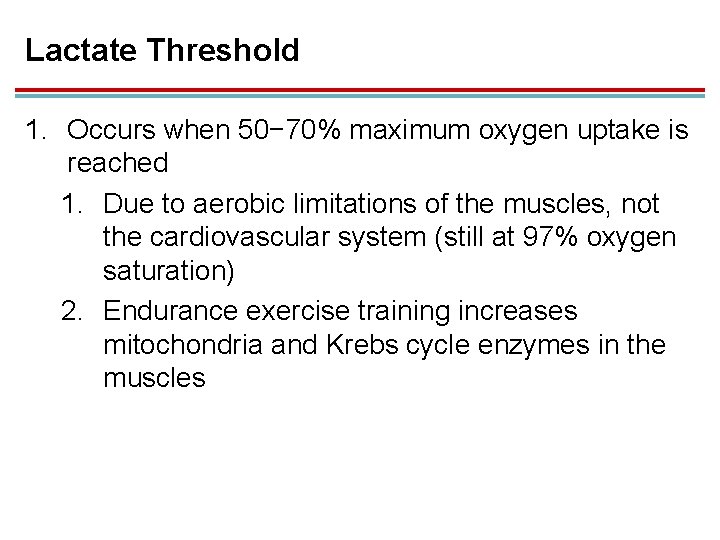
Lactate Threshold 1. Occurs when 50− 70% maximum oxygen uptake is reached 1. Due to aerobic limitations of the muscles, not the cardiovascular system (still at 97% oxygen saturation) 2. Endurance exercise training increases mitochondria and Krebs cycle enzymes in the muscles
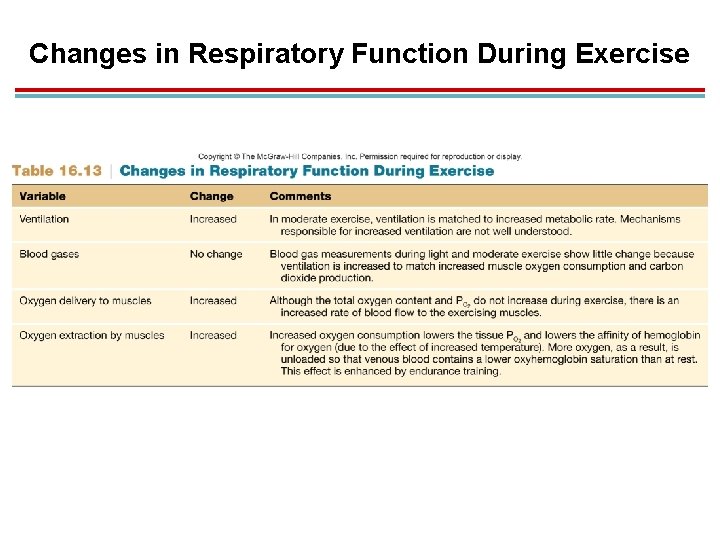
Changes in Respiratory Function During Exercise
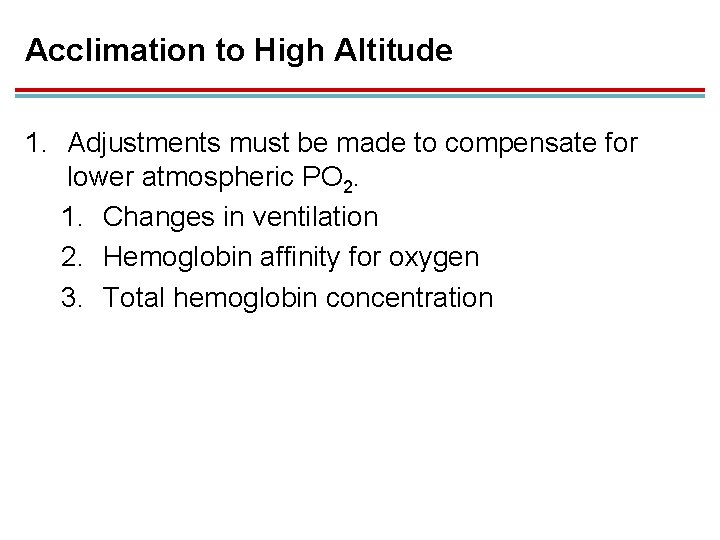
Acclimation to High Altitude 1. Adjustments must be made to compensate for lower atmospheric PO 2. 1. Changes in ventilation 2. Hemoglobin affinity for oxygen 3. Total hemoglobin concentration
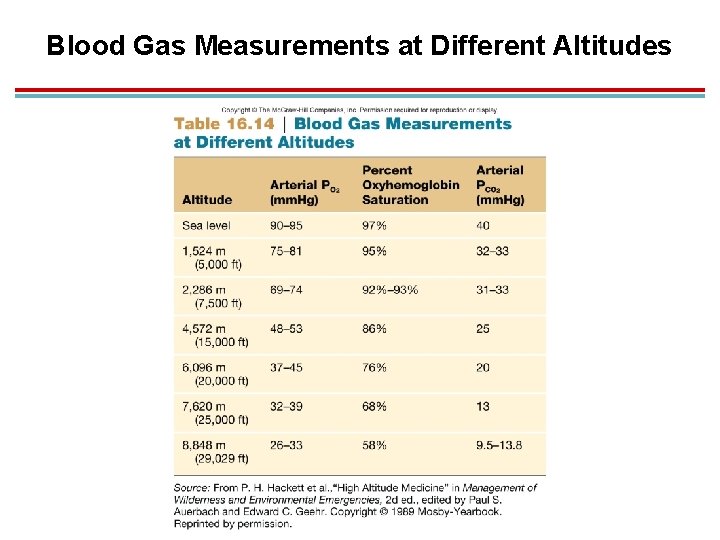
Blood Gas Measurements at Different Altitudes
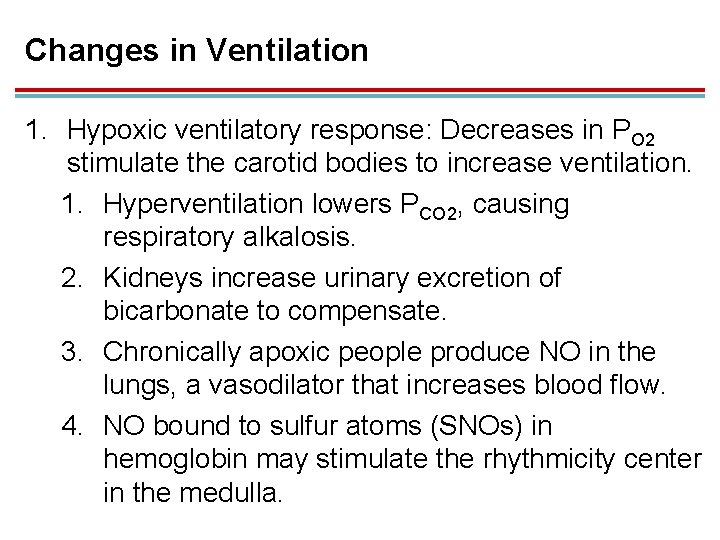
Changes in Ventilation 1. Hypoxic ventilatory response: Decreases in PO 2 stimulate the carotid bodies to increase ventilation. 1. Hyperventilation lowers PCO 2, causing respiratory alkalosis. 2. Kidneys increase urinary excretion of bicarbonate to compensate. 3. Chronically apoxic people produce NO in the lungs, a vasodilator that increases blood flow. 4. NO bound to sulfur atoms (SNOs) in hemoglobin may stimulate the rhythmicity center in the medulla.
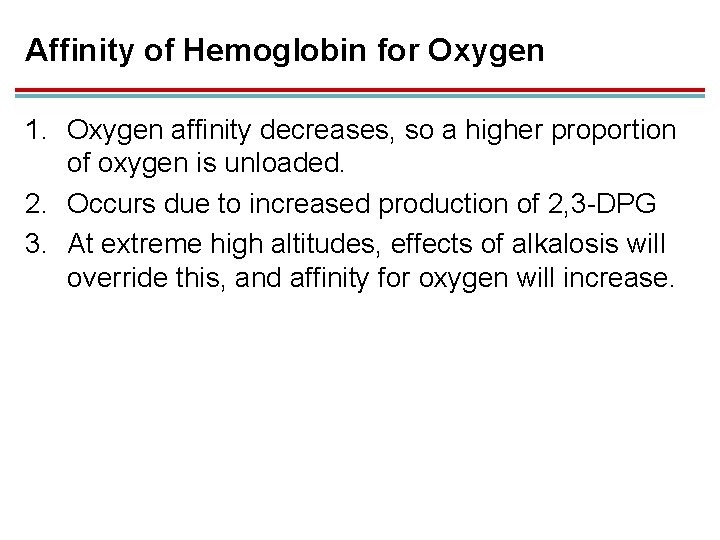
Affinity of Hemoglobin for Oxygen 1. Oxygen affinity decreases, so a higher proportion of oxygen is unloaded. 2. Occurs due to increased production of 2, 3 -DPG 3. At extreme high altitudes, effects of alkalosis will override this, and affinity for oxygen will increase.
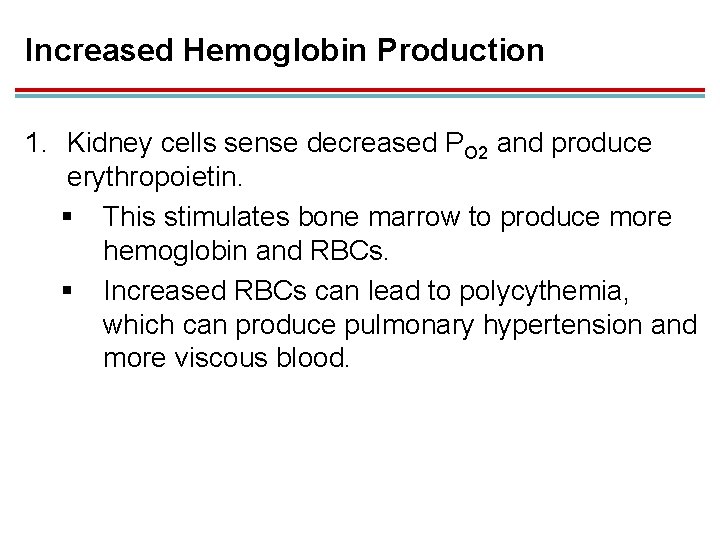
Increased Hemoglobin Production 1. Kidney cells sense decreased PO 2 and produce erythropoietin. § This stimulates bone marrow to produce more hemoglobin and RBCs. § Increased RBCs can lead to polycythemia, which can produce pulmonary hypertension and more viscous blood.
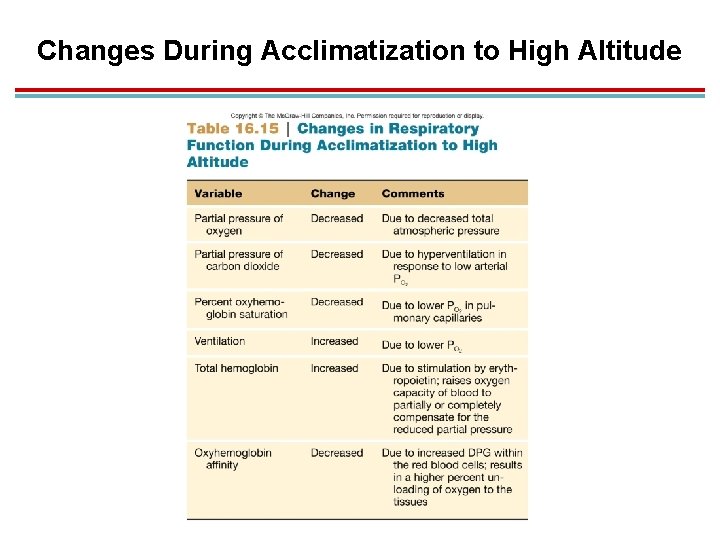
Changes During Acclimatization to High Altitude
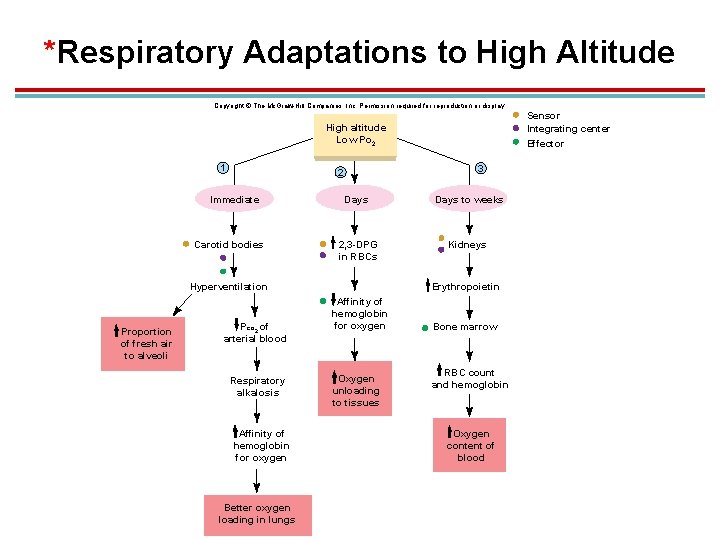
*Respiratory Adaptations to High Altitude Copyright © The Mc. Graw-Hill Companies, Inc. Permission required for reproduction or display. Sensor Integrating center Effector High altitude Low Po 2 1 3 2 Immediate Carotid bodies Days to weeks 2, 3 -DPG in RBCs Kidneys Hyperventilation Proportion of fresh air to alveoli Pco 2 of arterial blood Respiratory alkalosis Affinity of hemoglobin for oxygen Better oxygen loading in lungs Erythropoietin Affinity of hemoglobin for oxygen Oxygen unloading to tissues Bone marrow RBC count and hemoglobin Oxygen content of blood
Respiratory zone of the respiratory system
Respiratory system circulatory system digestive system
Larynx respiratory system
Part of the respiratory system
Part of respiratory system
Tiny air sacs at the end of the bronchioles
Circulatory system and respiratory system work together
Chapter 7 cengage
Bronchide
Respiratory perfusion
Chapter 15 lesson 4
Chapter 7 the respiratory system labeling exercises
Chapter 34 section 1 the circulatory system
Respiratory system organs
Alveolar ducts and alveoli
Chapter 17 respiratory system workbook answers
Bozeman respiratory system
Unit 9 respiratory system
Diagnostic test of respiratory system
What is the respiratory system
Respiratory system coloring page
Respiratory system purpose
Conclusion of the respiratory system
Jobs of respiratory system
Interesting facts about respiratory system
5 functions of the respiratory system
How to draw the respiratory system
What is the major function of the respiratory system
Respiratory system diagram unlabeled
Respiratory system vocabulary
Breathing mechanism
Kylie knh
Stretched nostrils
History taking of patient
Upper and lower respiratory tract
Trachea function
Sharks respiration
The path of air through the respiratory system
Fish respiratory system
Phylum arthropoda marine
Exhale respiratory system
Polychaeta respiratory system
What is the conducting zone of the respiratory system
Respiratory system of rabbit
The respiratory system just wasting away answer key
Pharynx histology
Anatomical directions of frog
Figure 13-1
Pig anatomy
Fetal pig circulatory system
Respiratory system flow chart
Interesting facts about respiratory system
Precocial species
Vocal sac opening frog
Respiratory zone
Respiratory system meaning
Learning objectives of respiratory system
Respiratory system introduction
Functional anatomy of the respiratory system
Systemic examination of respiratory system
Stress and respiratory system
The respiratory system
Starfish
Pharmacology of drugs acting on respiratory system
Circulatory and respiratory system working together
Parts of the air
Brainpop asthma
The respiratory system
Respiratory system pictures
How we breathe oxygen
Aeoli lungs
Minute respiratory volume
Contoh terminologi
The respiratory system
Pathway of air in respiratory system
Taking care of your respiratory system
Which organs are involved in respiratory system
Respiratory system
Primary secondary and tertiary bronchi
Aortic impression lung
Learning objectives of respiratory system
Order of inhalation
Respiratory buffer
Respiratory system nasal cavity
Development of respiratory system