AP Chemistry Electronic Structure of Atoms electronic structure
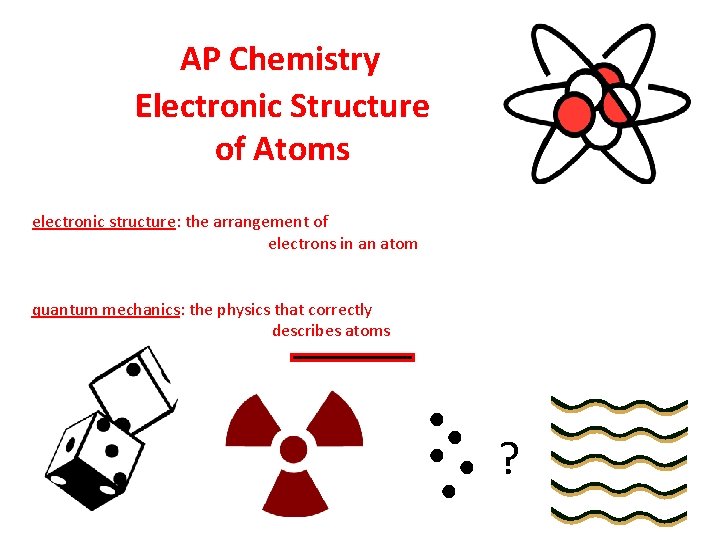
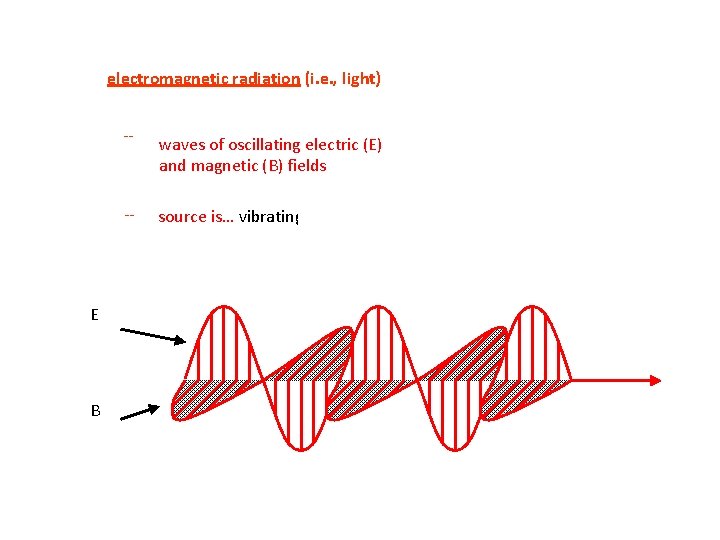
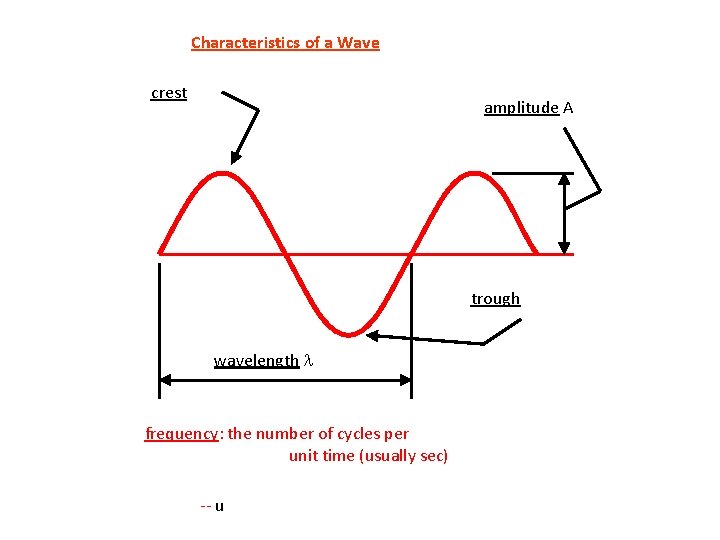
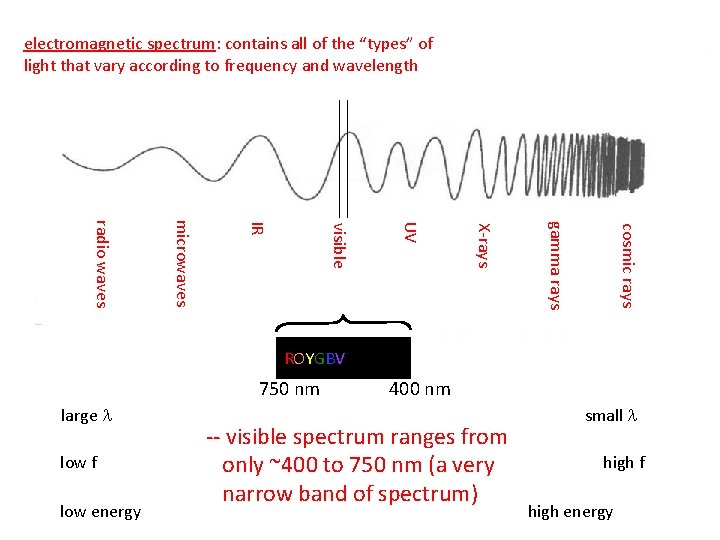
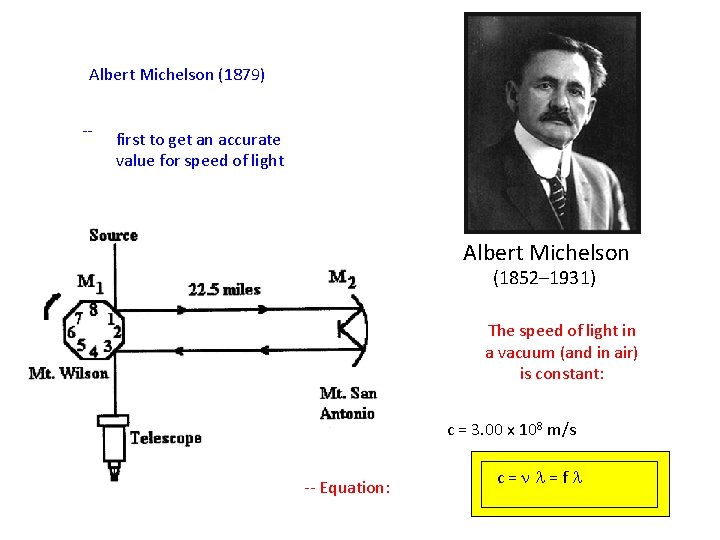
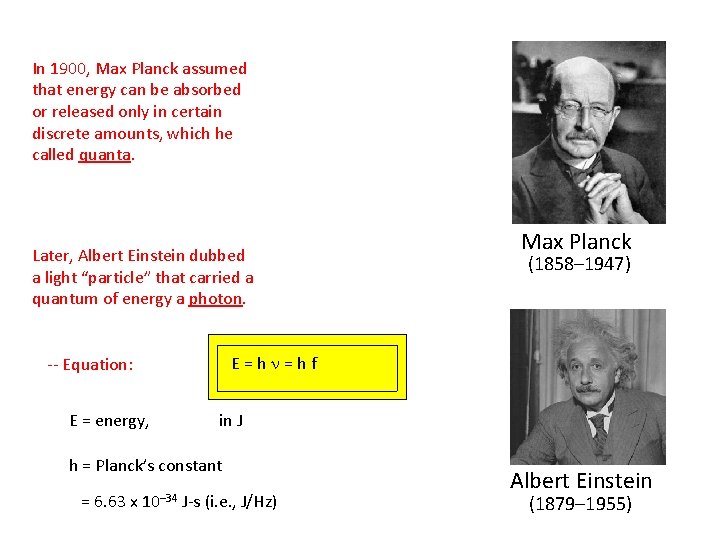
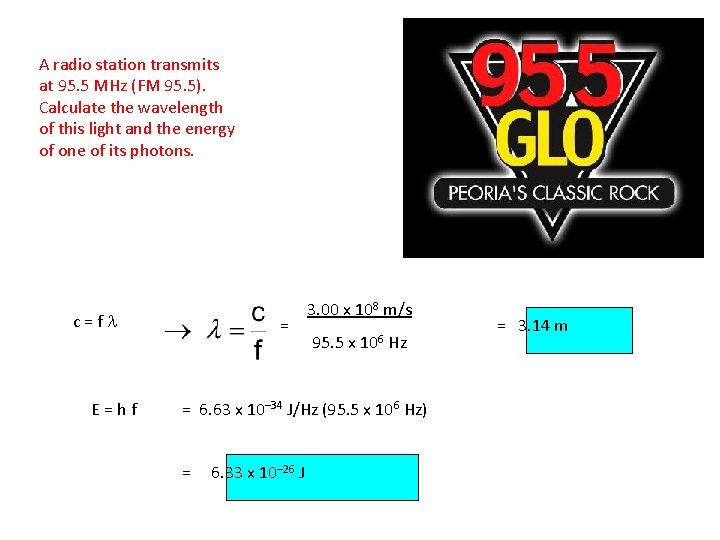
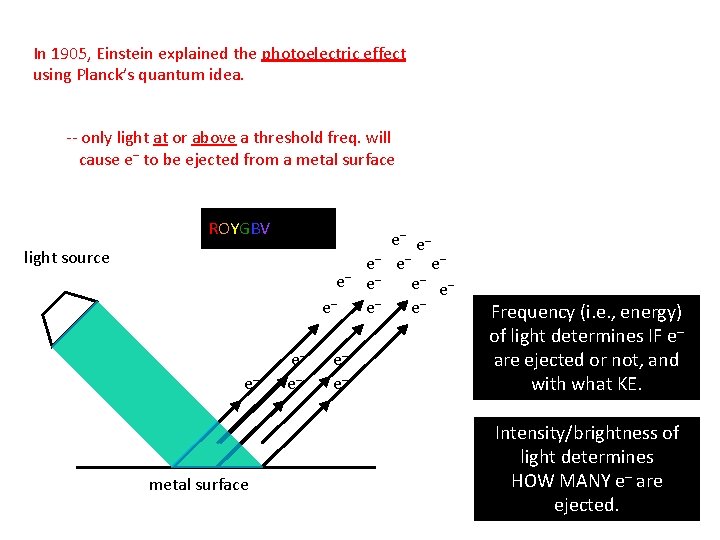
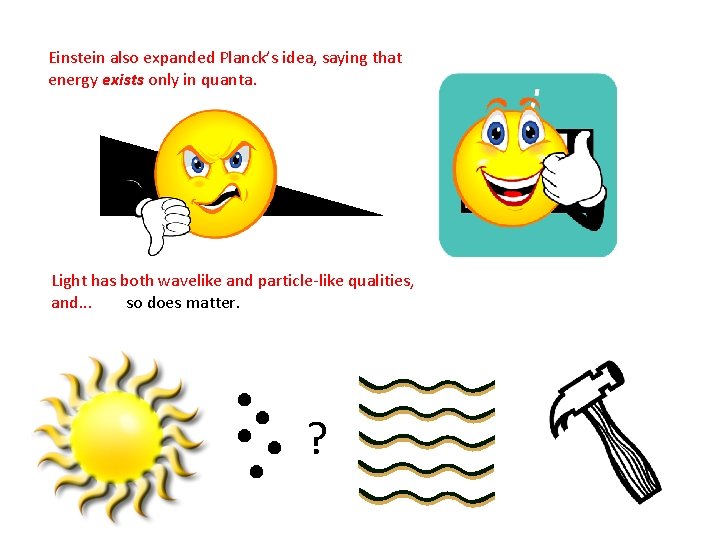
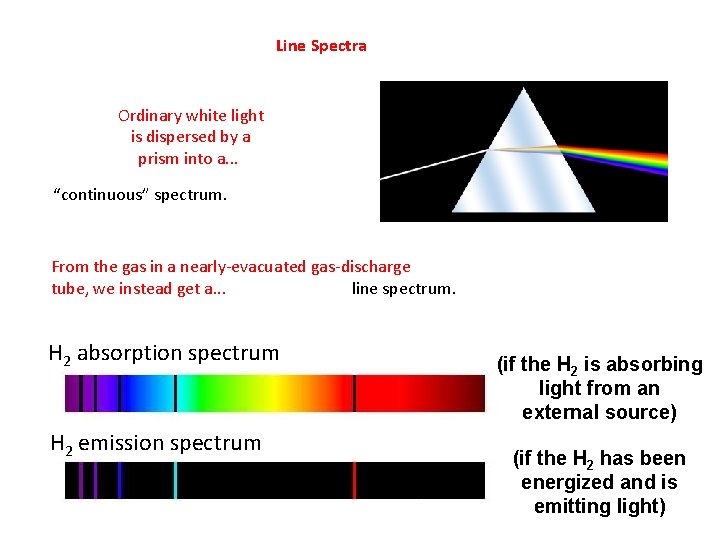
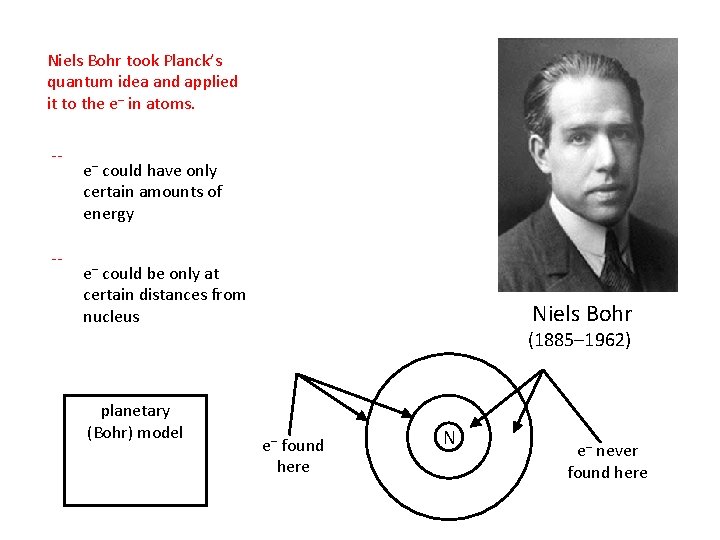
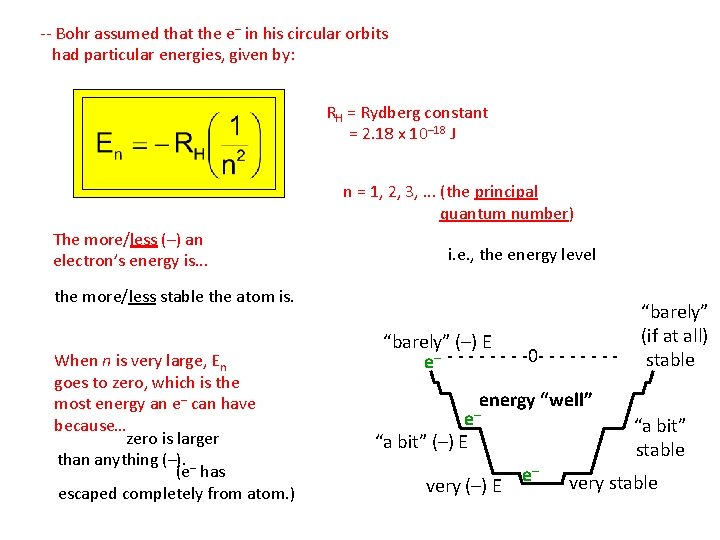
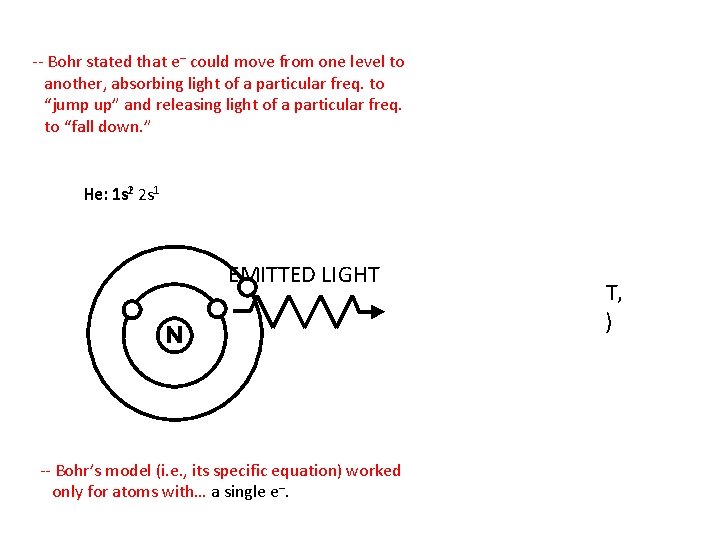
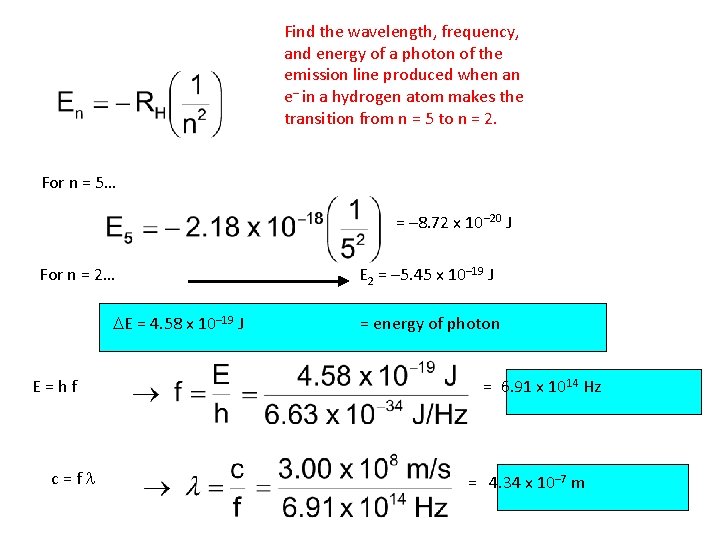
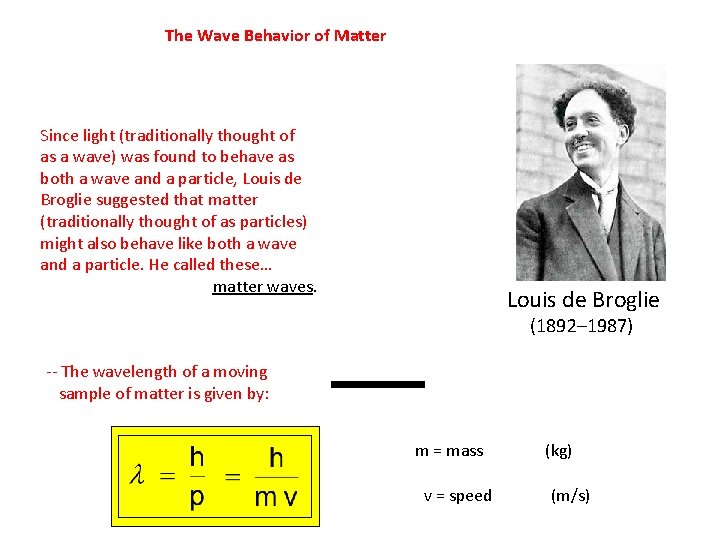
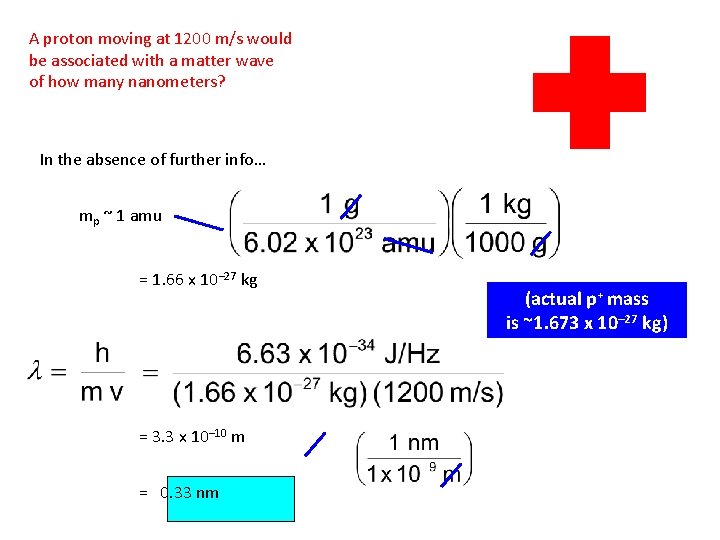
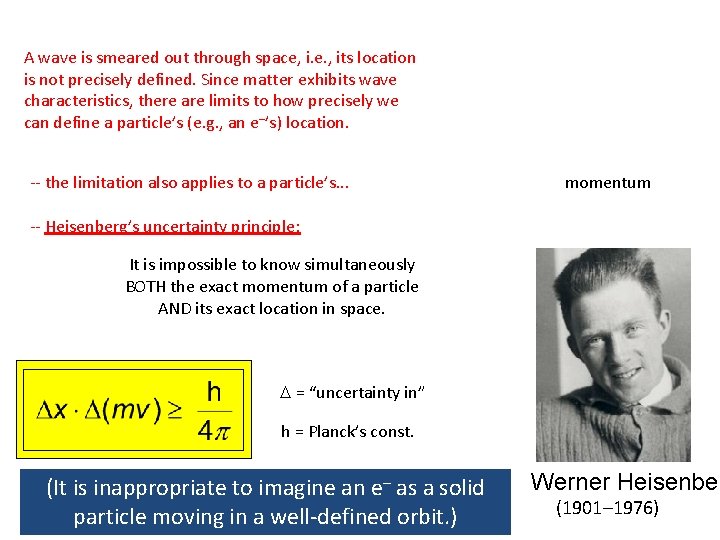
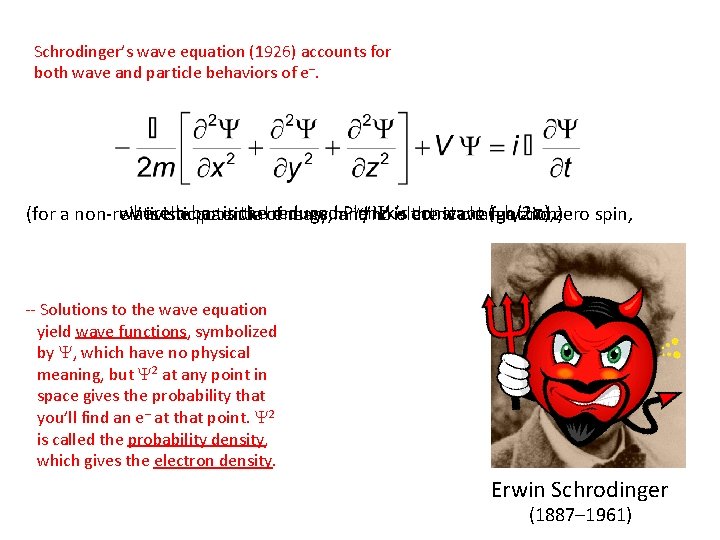
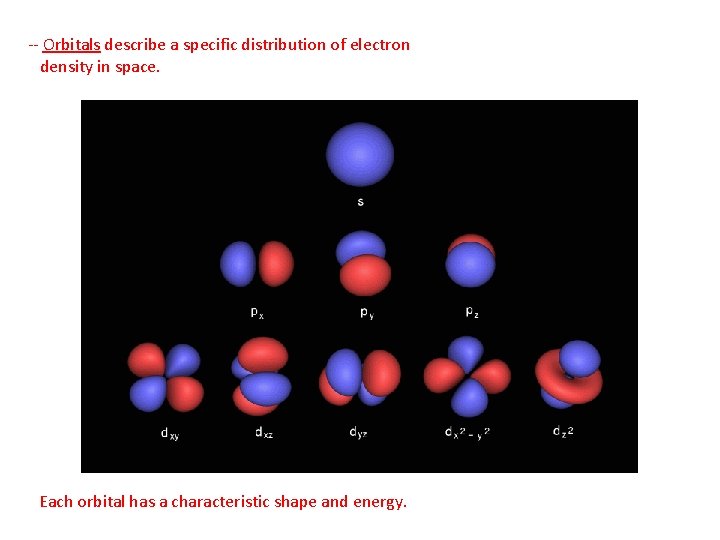
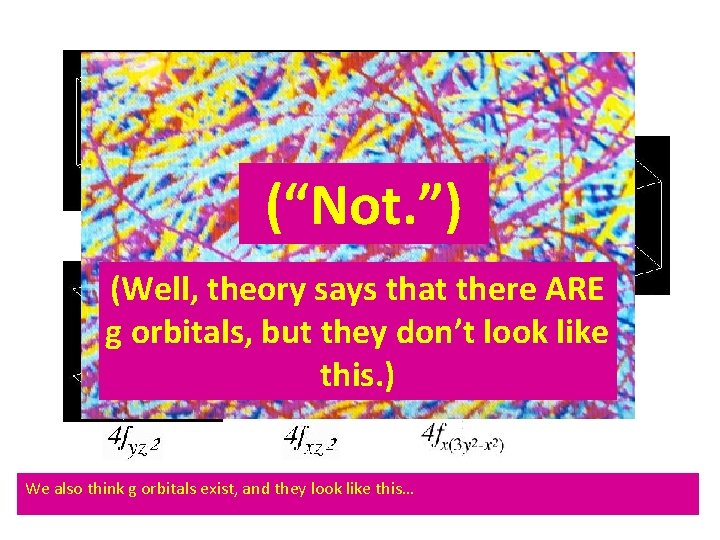
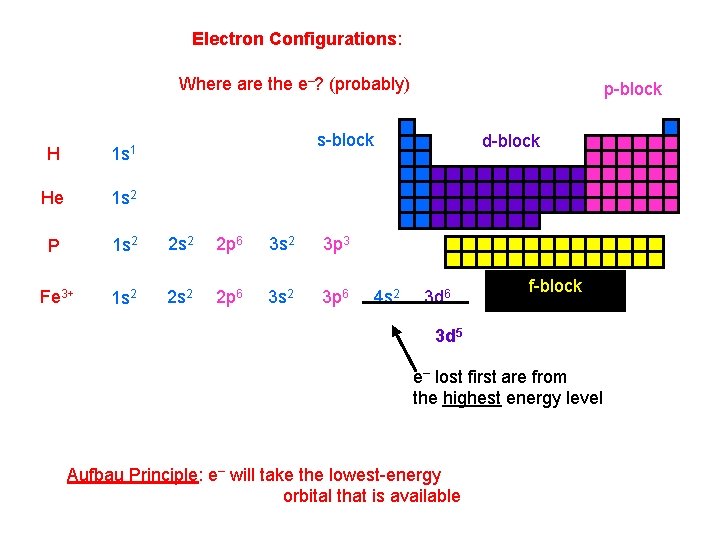
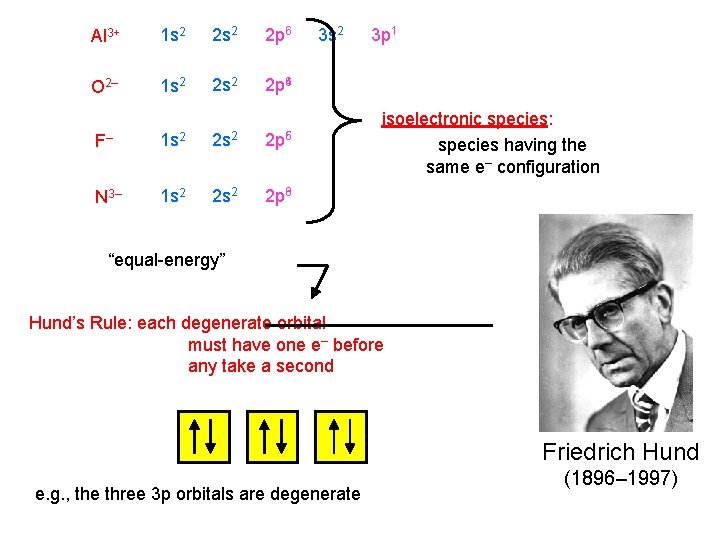
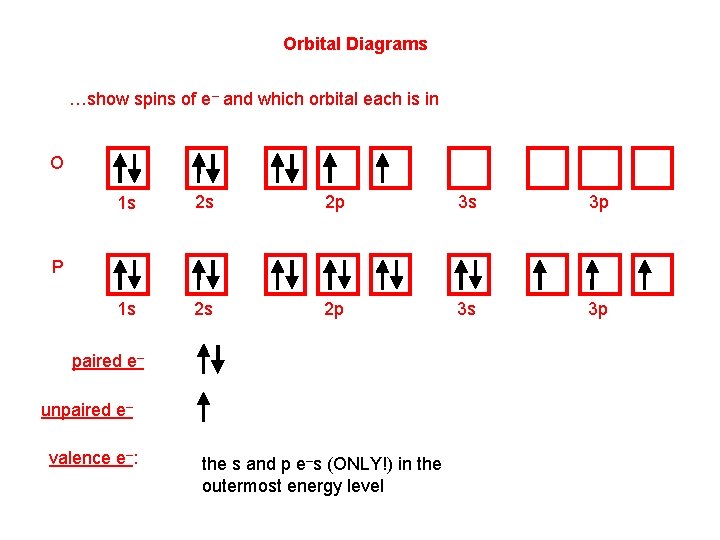
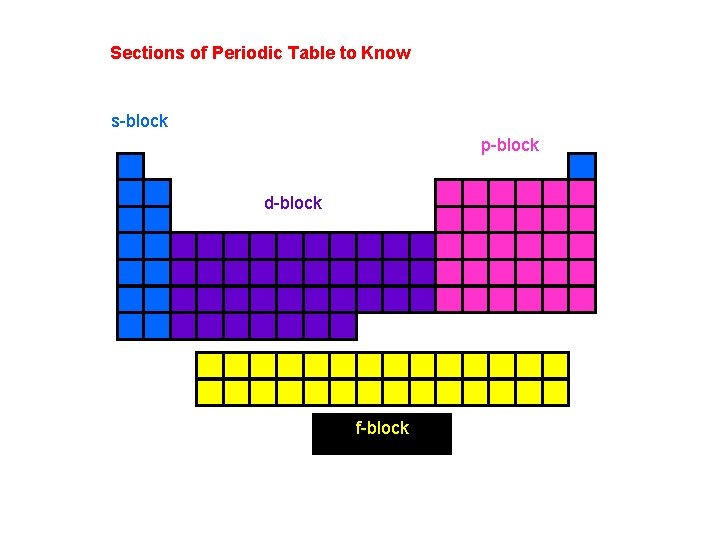
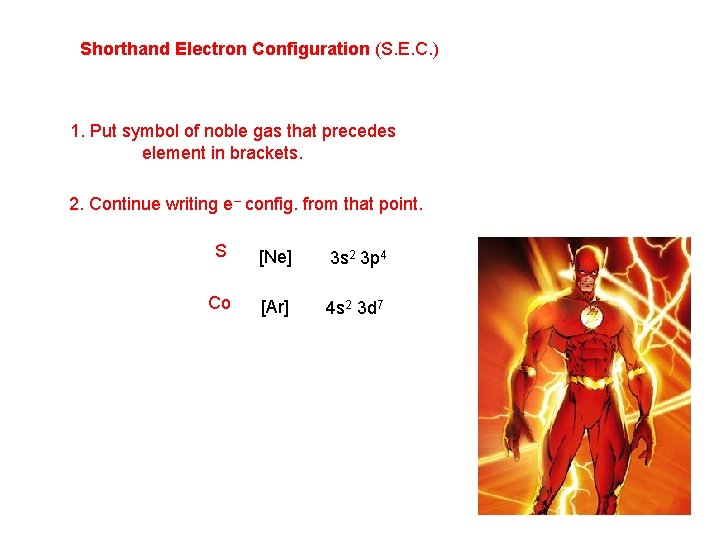
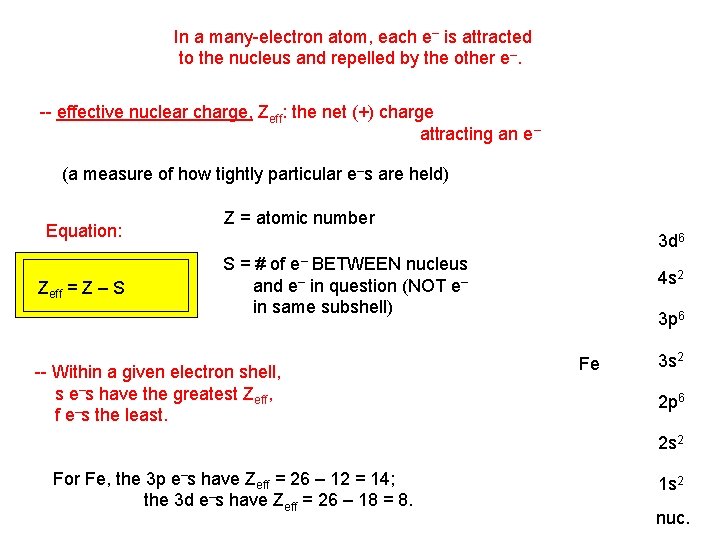
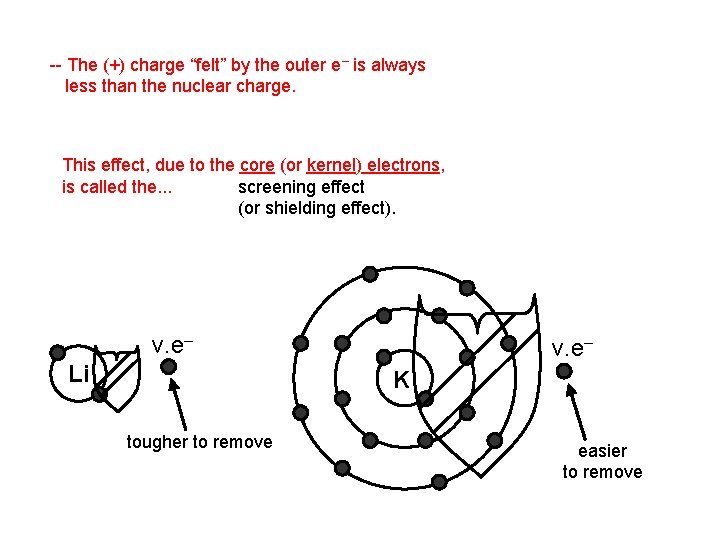
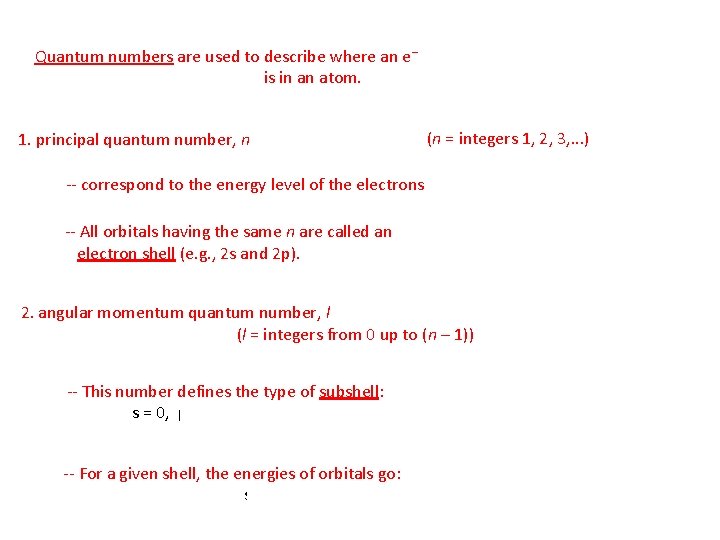
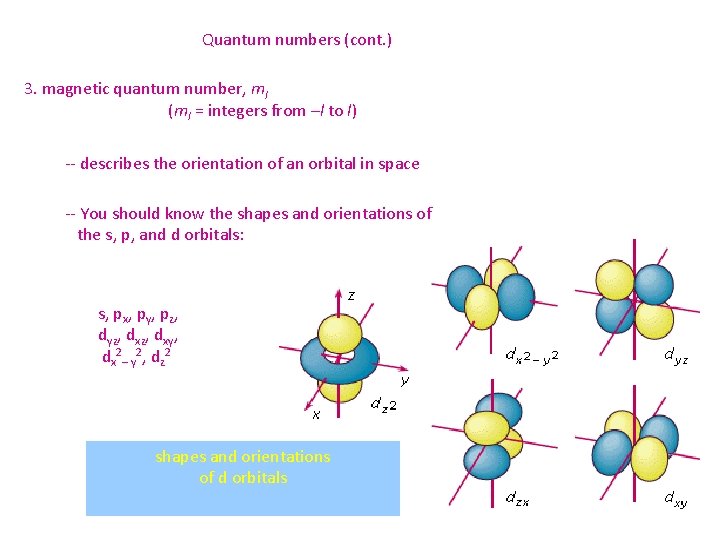
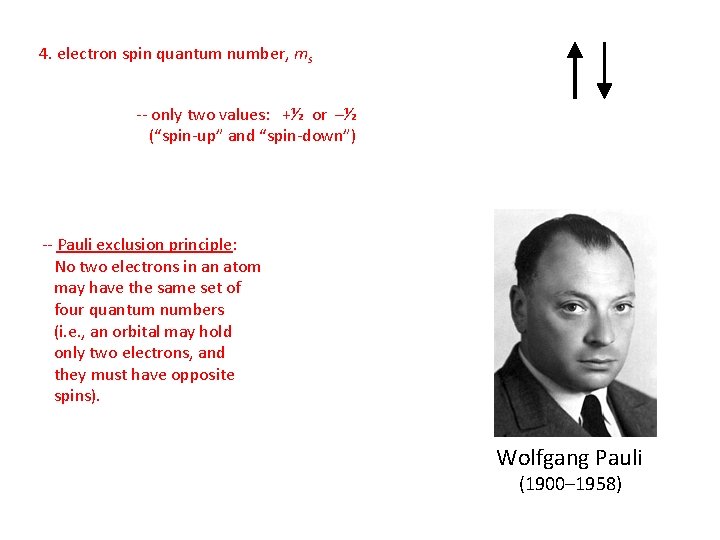
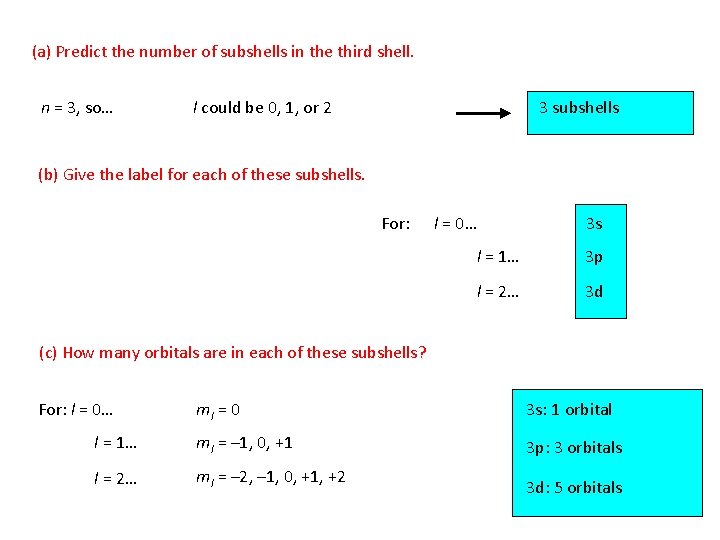
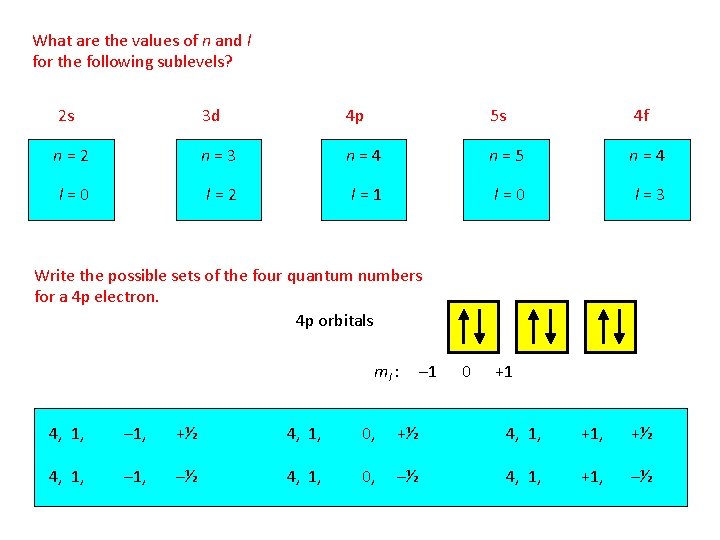
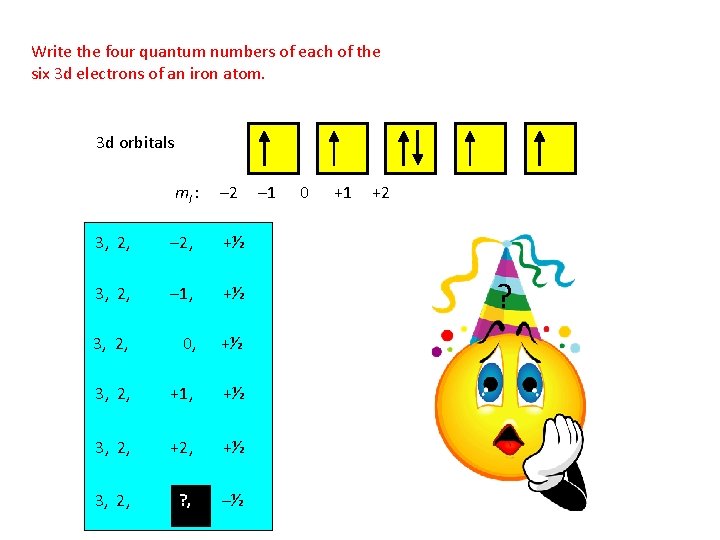
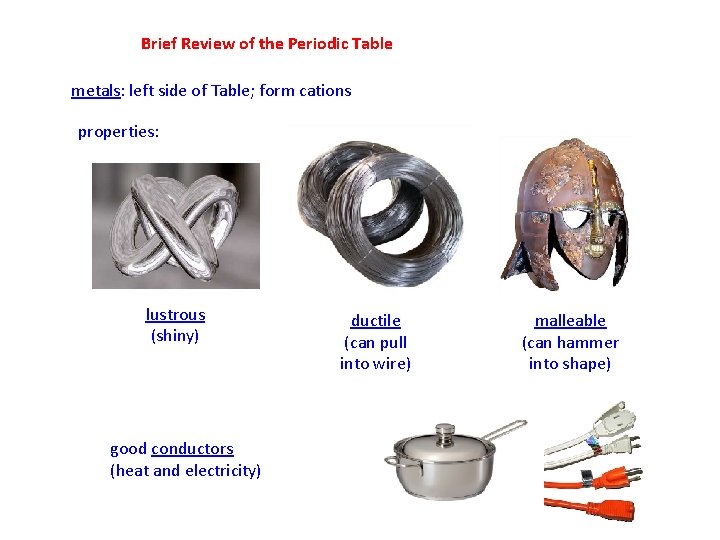
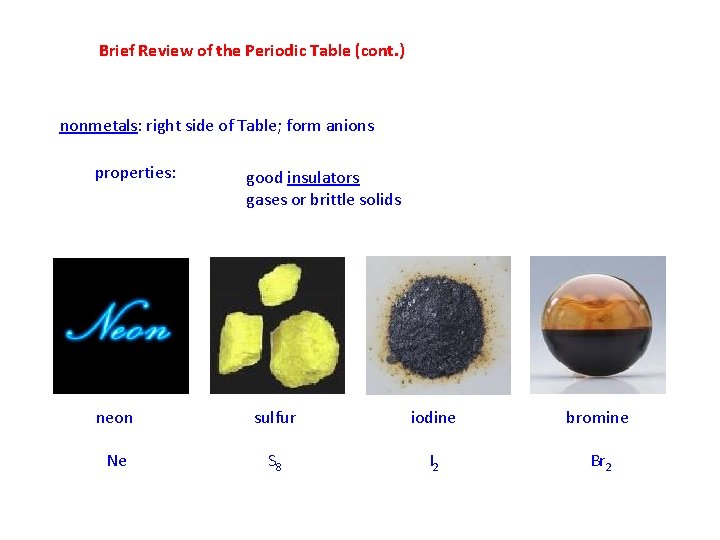
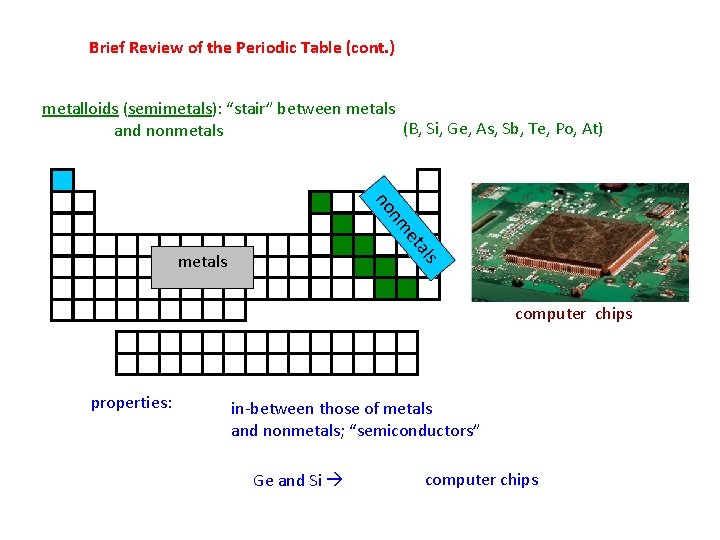
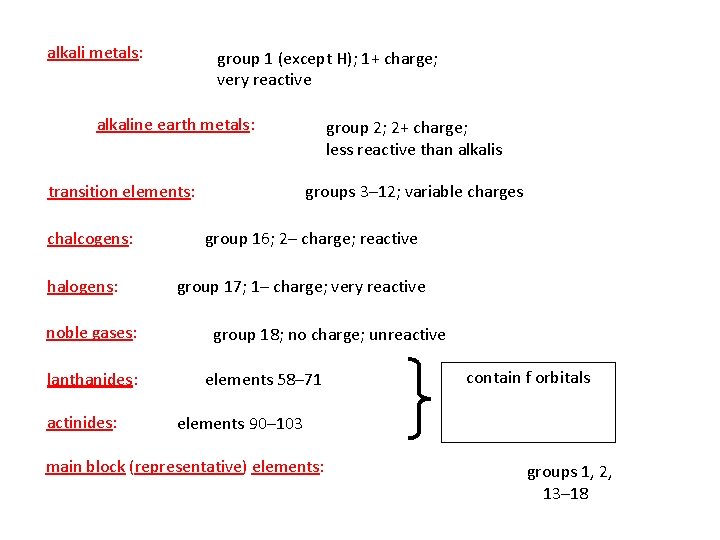
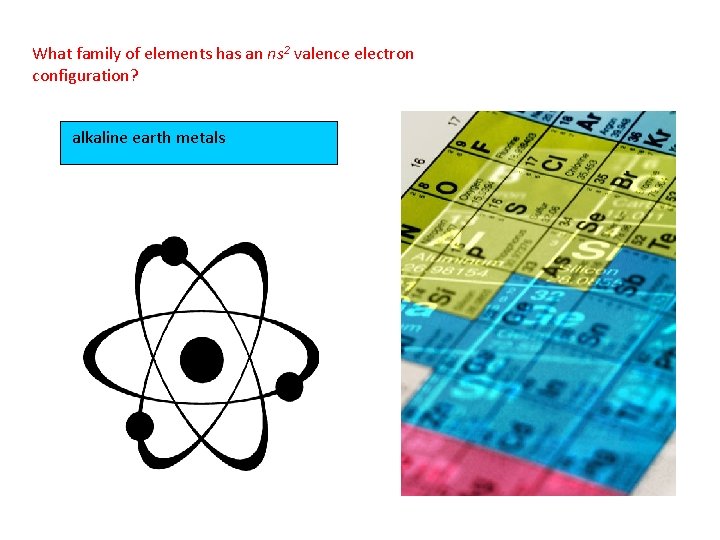
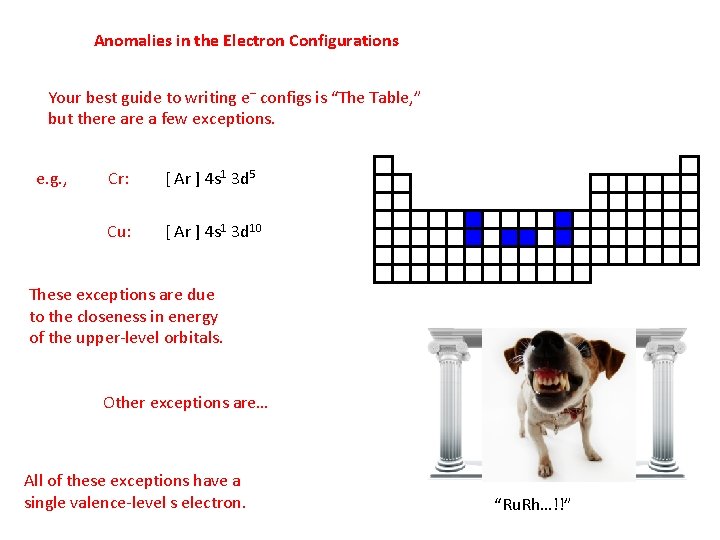
- Slides: 39
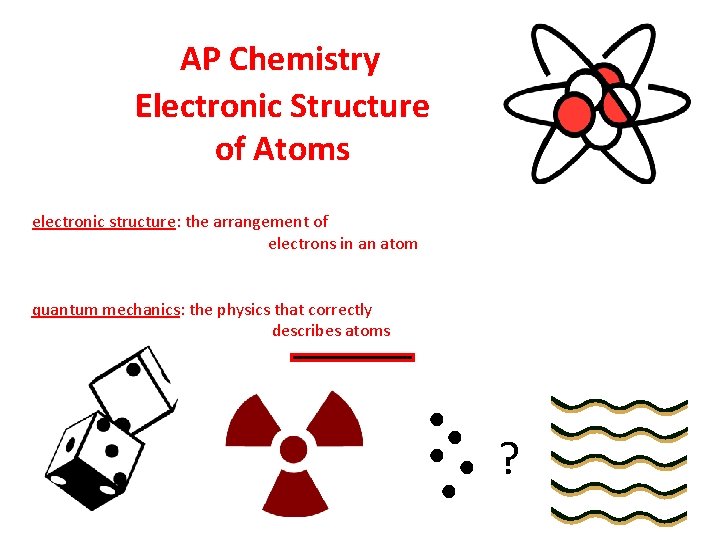
AP Chemistry Electronic Structure of Atoms electronic structure: the arrangement of electrons in an atom quantum mechanics: the physics that correctly describes atoms ?
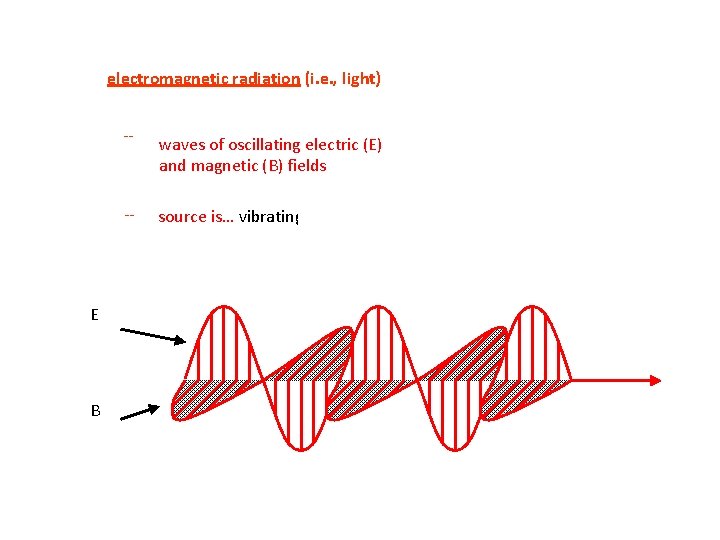
electromagnetic radiation (i. e. , light) E B -- waves of oscillating electric (E) and magnetic (B) fields -- source is… vibrating electric charges
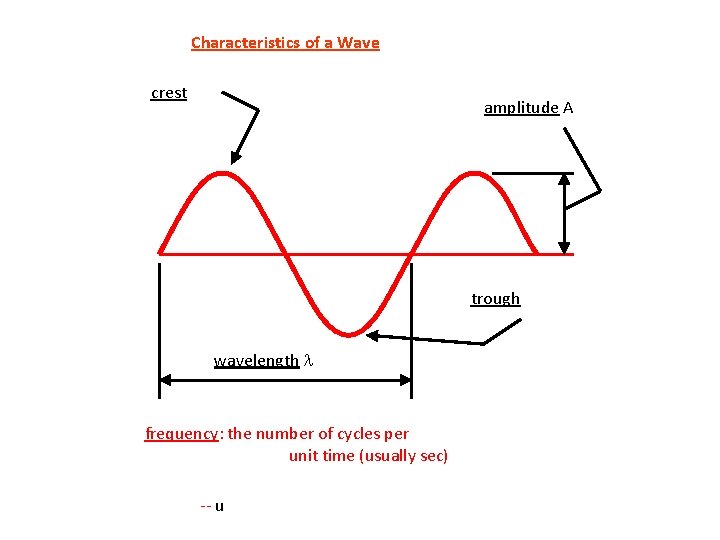
Characteristics of a Wave crest amplitude A trough wavelength l frequency: the number of cycles per unit time (usually sec) -- unit is Hz, or s– 1
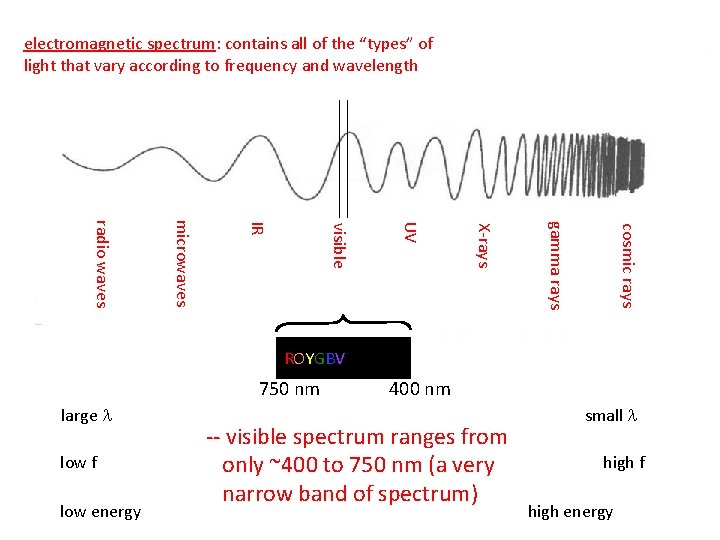
electromagnetic spectrum: contains all of the “types” of light that vary according to frequency and wavelength cosmic rays gamma rays X-rays UV visible IR microwaves radio waves ROYGBV 750 nm large l low f low energy 400 nm -- visible spectrum ranges from only ~400 to 750 nm (a very narrow band of spectrum) small l high f high energy
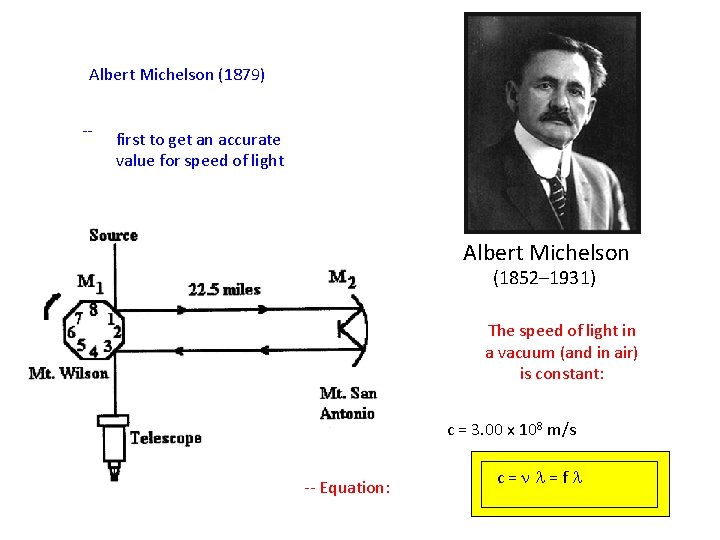
Albert Michelson (1879) -- first to get an accurate value for speed of light Albert Michelson (1852– 1931) The speed of light in a vacuum (and in air) is constant: c = 3. 00 x 108 m/s -- Equation: c=nl=fl
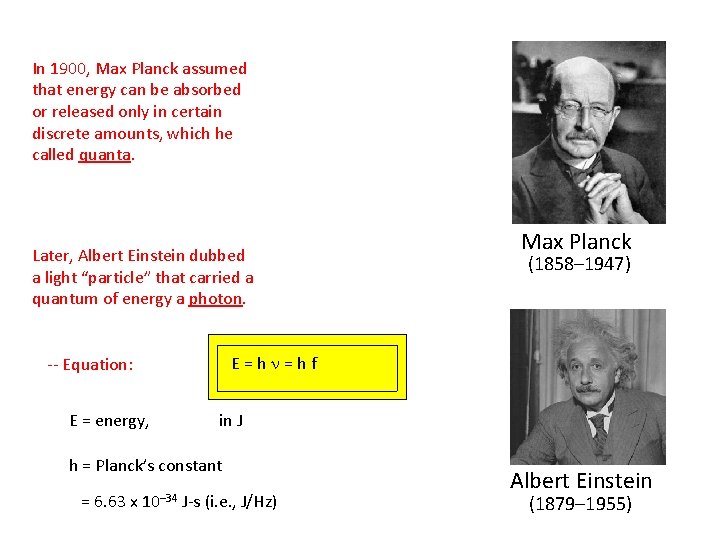
In 1900, Max Planck assumed that energy can be absorbed or released only in certain discrete amounts, which he called quanta. Later, Albert Einstein dubbed a light “particle” that carried a quantum of energy a photon. (1858– 1947) E=hn=hf -- Equation: E = energy, in J h = Planck’s constant = 6. 63 x Max Planck 10– 34 J-s (i. e. , J/Hz) Albert Einstein (1879– 1955)
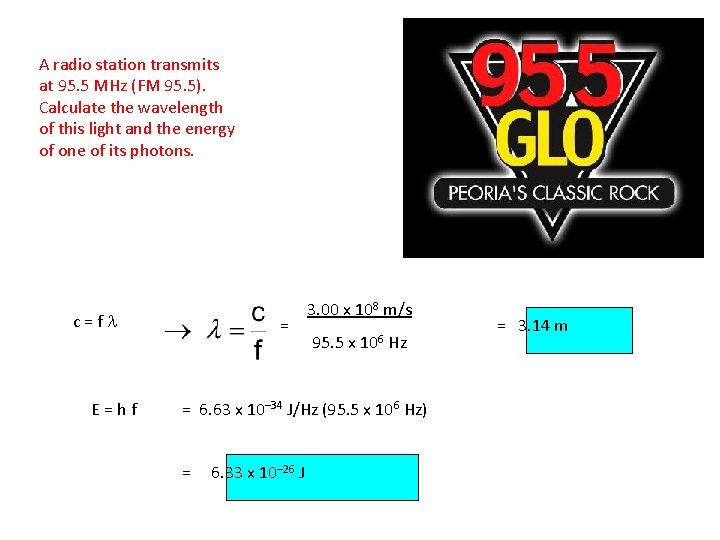
A radio station transmits at 95. 5 MHz (FM 95. 5). Calculate the wavelength of this light and the energy of one of its photons. c=fl E=hf = 3. 00 x 108 m/s 95. 5 x 106 Hz = 6. 63 x 10– 34 J/Hz (95. 5 x 106 Hz) = 6. 33 x 10– 26 J = 3. 14 m
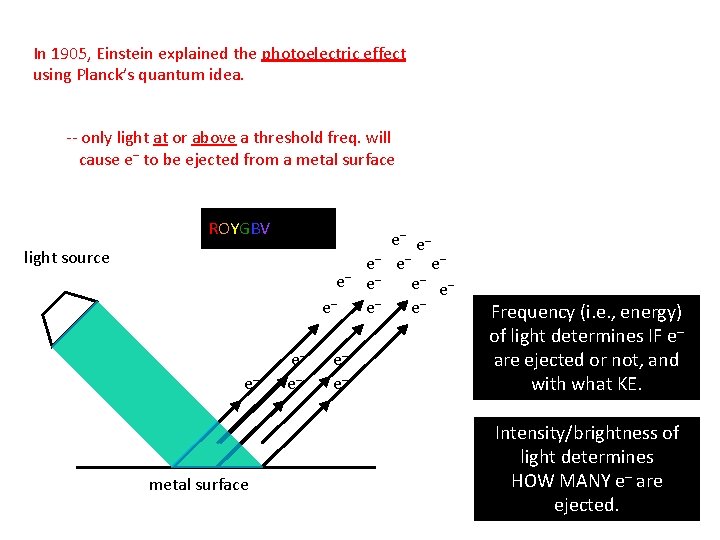
In 1905, Einstein explained the photoelectric effect using Planck’s quantum idea. -- only light at or above a threshold freq. will cause e– to be ejected from a metal surface ROYGBV light source e– e– e– metal surface e– e– e– e– Frequency (i. e. , energy) of light determines IF e– are ejected or not, and with what KE. Intensity/brightness of light determines HOW MANY e– are ejected.
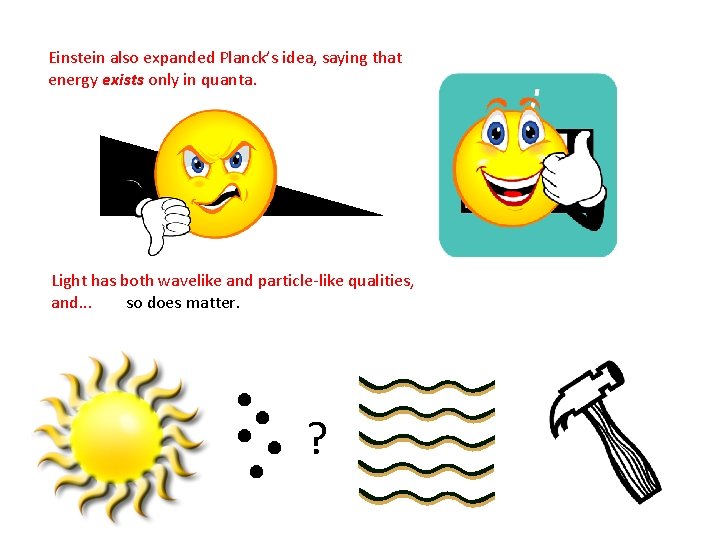
Einstein also expanded Planck’s idea, saying that energy exists only in quanta. Light has both wavelike and particle-like qualities, and. . . so does matter. ?
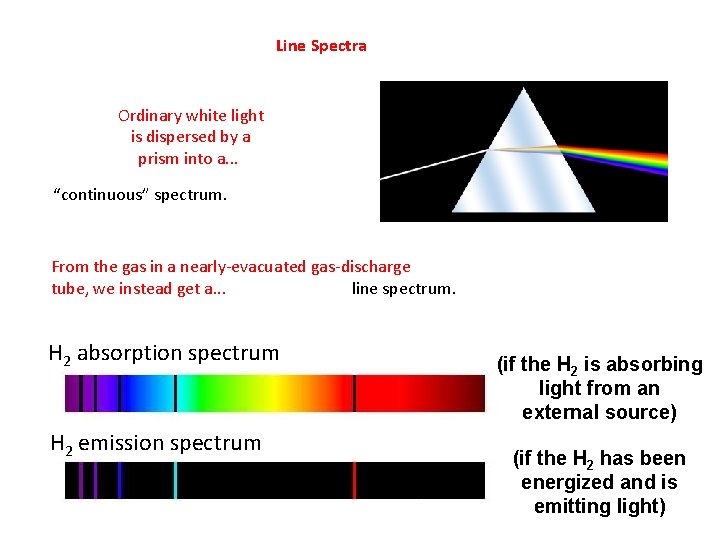
Line Spectra Ordinary white light is dispersed by a prism into a. . . “continuous” spectrum. From the gas in a nearly-evacuated gas-discharge tube, we instead get a. . . line spectrum. H 2 absorption spectrum H 2 emission spectrum (if the H 2 is absorbing light from an external source) (if the H 2 has been energized and is emitting light)
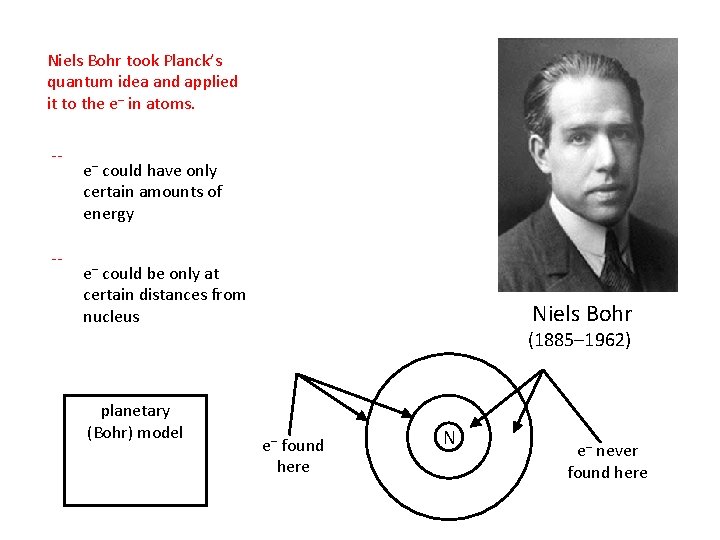
Niels Bohr took Planck’s quantum idea and applied it to the e– in atoms. -- -- e– could have only certain amounts of energy e– could be only at certain distances from nucleus planetary (Bohr) model Niels Bohr (1885– 1962) e– found here N e– never found here
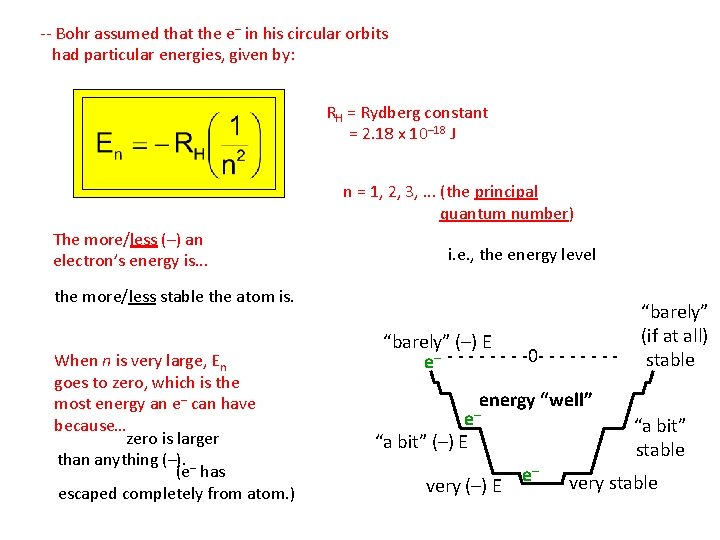
-- Bohr assumed that the e– in his circular orbits had particular energies, given by: RH = Rydberg constant = 2. 18 x 10– 18 J n = 1, 2, 3, . . . (the principal quantum number) The more/less (–) an electron’s energy is. . . i. e. , the energy level the more/less stable the atom is. When n is very large, En goes to zero, which is the most energy an e– can have because… zero is larger than anything (–). – (e has escaped completely from atom. ) “barely” (–) E e– - - - -0 - - - - “barely” (if at all) stable energy “well” e– “a bit” (–) E very (–) E e– “a bit” stable very stable
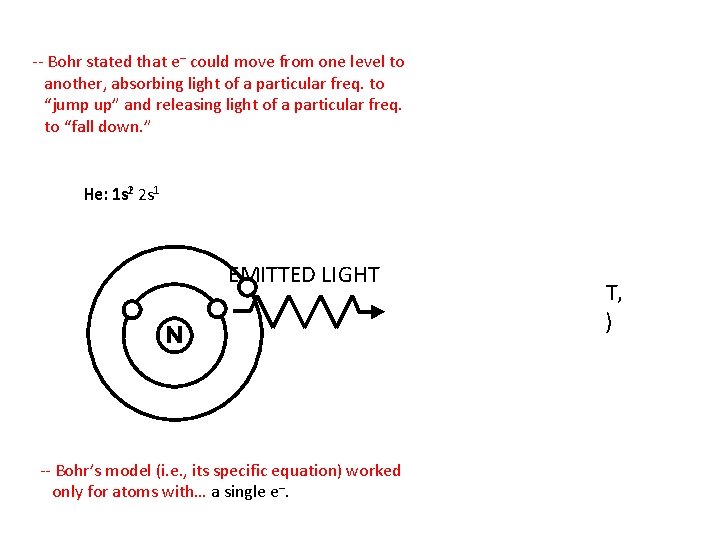
-- Bohr stated that e– could move from one level to another, absorbing light of a particular freq. to “jump up” and releasing light of a particular freq. to “fall down. ” He: 1 s 12 2 s 1 ENERGY EMITTED LIGHT -- Bohr’s model (i. e. , its specific equation) worked only for atoms with… a single e–. (HEAT, LIGHT, ELEC. , ETC. )
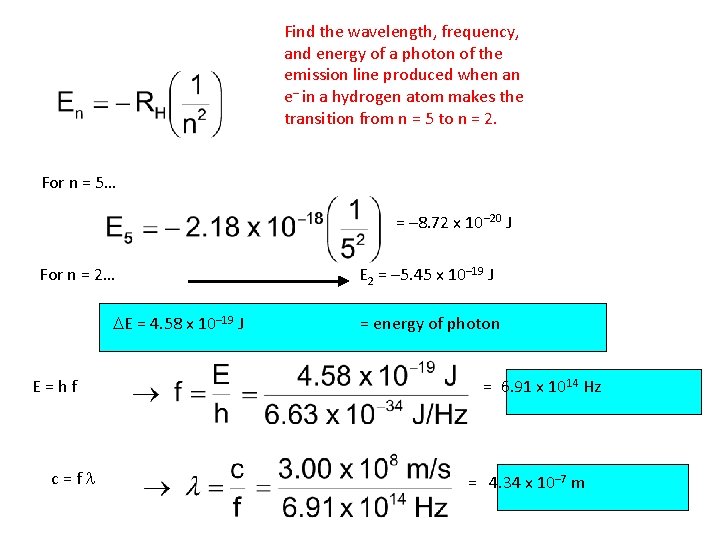
Find the wavelength, frequency, and energy of a photon of the emission line produced when an e– in a hydrogen atom makes the transition from n = 5 to n = 2. For n = 5… = – 8. 72 x 10– 20 J For n = 2… DE = 4. 58 x 10– 19 J E=hf c=fl E 2 = – 5. 45 x 10– 19 J = energy of photon = 6. 91 x 1014 Hz = 4. 34 x 10– 7 m
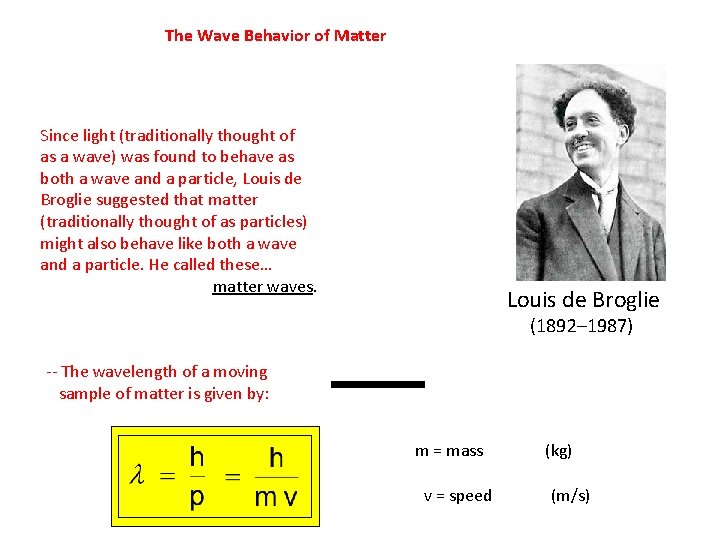
The Wave Behavior of Matter Since light (traditionally thought of as a wave) was found to behave as both a wave and a particle, Louis de Broglie suggested that matter (traditionally thought of as particles) might also behave like both a wave and a particle. He called these… matter waves. Louis de Broglie (1892– 1987) -- The wavelength of a moving sample of matter is given by: m = mass v = speed (kg) (m/s)
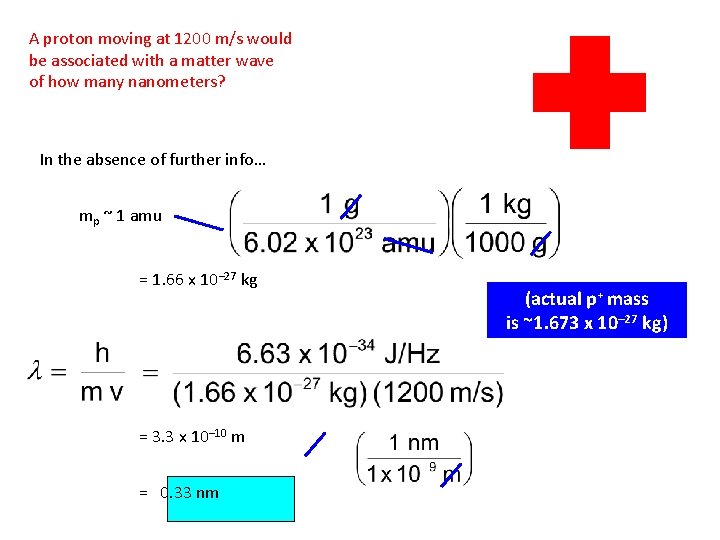
A proton moving at 1200 m/s would be associated with a matter wave of how many nanometers? In the absence of further info… mp ~ 1 amu = 1. 66 x 10– 27 kg = 3. 3 x 10– 10 m = 0. 33 nm (actual p+ mass is ~1. 673 x 10– 27 kg)
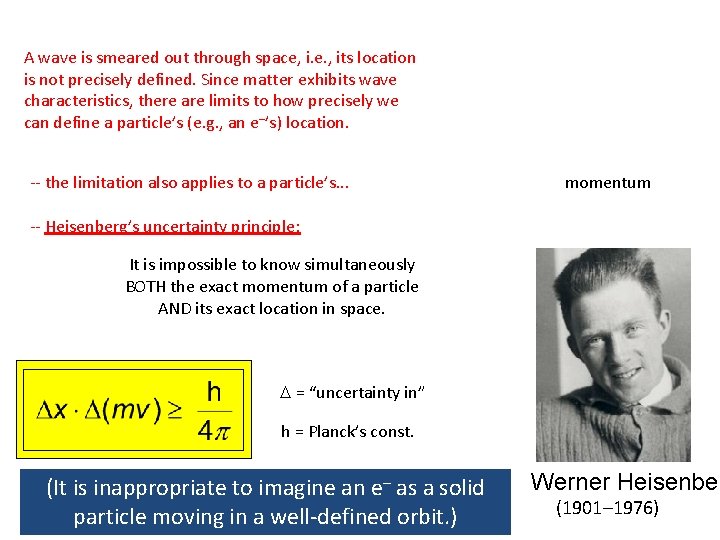
A wave is smeared out through space, i. e. , its location is not precisely defined. Since matter exhibits wave characteristics, there are limits to how precisely we can define a particle’s (e. g. , an e–’s) location. -- the limitation also applies to a particle’s. . . momentum -- Heisenberg’s uncertainty principle: It is impossible to know simultaneously BOTH the exact momentum of a particle AND its exact location in space. D = “uncertainty in” h = Planck’s const. (It is inappropriate to imagine an e– as a solid particle moving in a well-defined orbit. ) Werner Heisenber (1901– 1976)
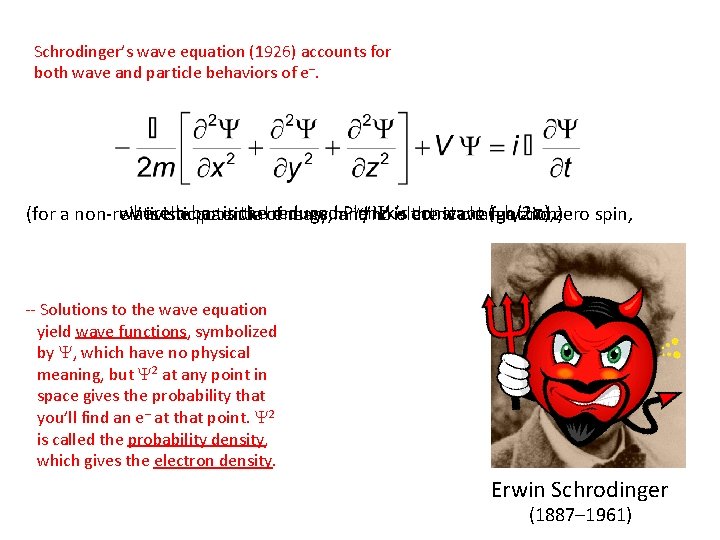
Schrodinger’s wave equation (1926) accounts for both wave and particle behaviors of e–. w/no where h-bar is theof reduced Planck’s constant (=h/2 p), V is the potential energy, and Y iselectric the wave function) (for a non-relativistic particle mass m charge and zero spin, -- Solutions to the wave equation yield wave functions, symbolized by Y, which have no physical meaning, but Y 2 at any point in space gives the probability that you’ll find an e– at that point. Y 2 is called the probability density, which gives the electron density. Erwin Schrodinger (1887– 1961)
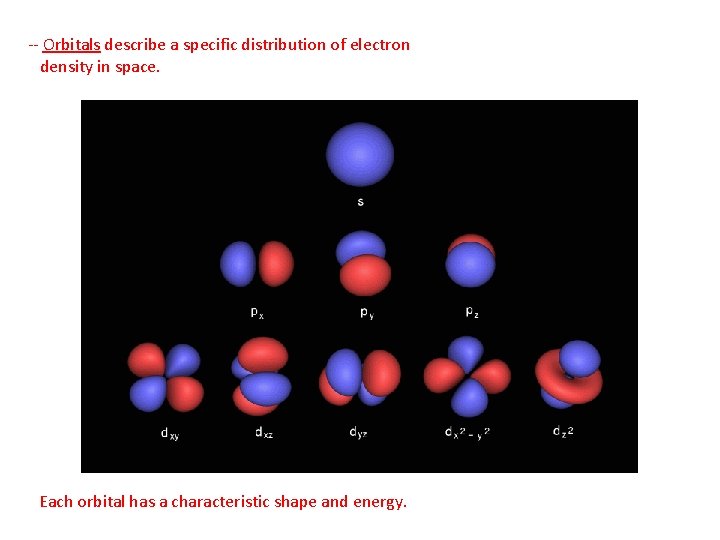
-- Orbitals describe a specific distribution of electron density in space. Each orbital has a characteristic shape and energy.
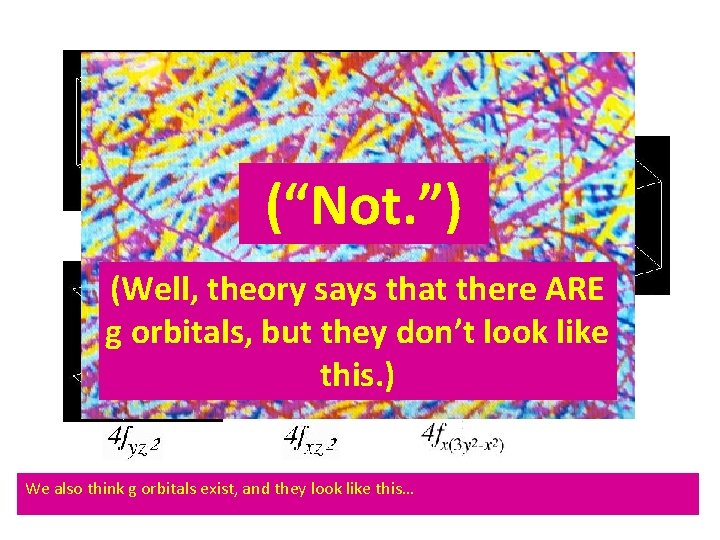
(“Not. ”) (Well, theory says that there ARE g orbitals, but they don’t look like this. ) We also g orbitals and they lookflike this… Thethink lanthanides andexist, actinides contain orbitals.
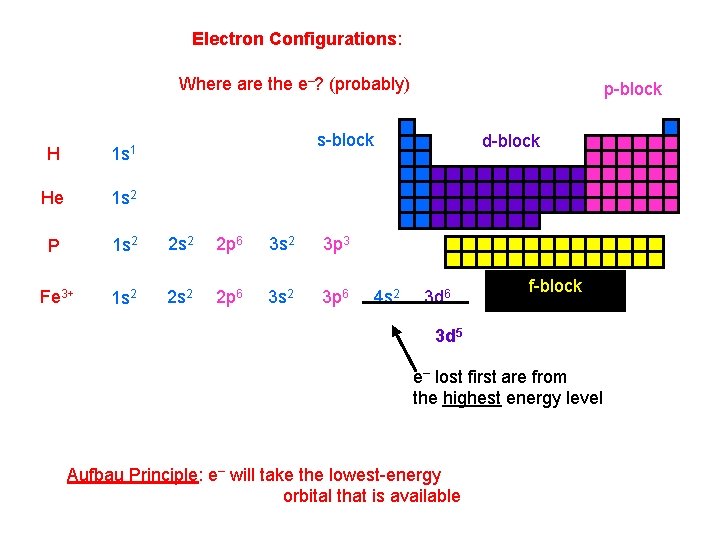
Electron Configurations: Where are the e–? (probably) p-block s-block H 1 s 1 He 1 s 2 P 1 s 2 2 p 6 3 s 2 3 p 3 Fe 3+ 1 s 2 2 p 6 3 s 2 3 p 6 d-block 4 s 2 3 d 6 f-block 3 d 5 e– lost first are from the highest energy level Aufbau Principle: e– will take the lowest-energy orbital that is available
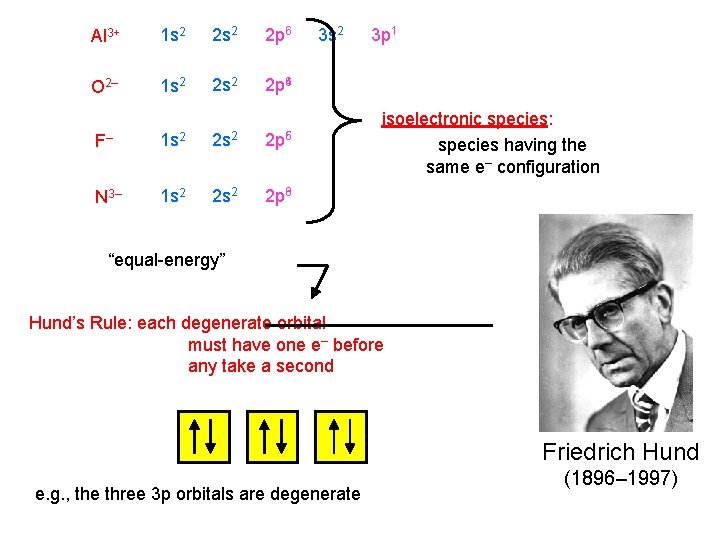
Al 3+ 1 s 2 2 p 6 O 2– 1 s 2 2 p 64 F– 1 s 2 2 p 56 N 3– 1 s 2 2 p 36 3 s 2 3 p 1 isoelectronic species: species having the same e– configuration “equal-energy” Hund’s Rule: each degenerate orbital must have one e– before any take a second Friedrich Hund e. g. , the three 3 p orbitals are degenerate (1896– 1997)
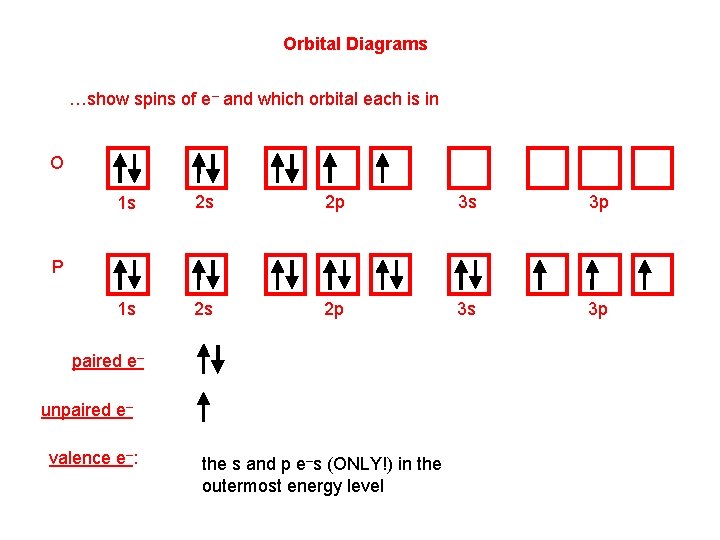
Orbital Diagrams …show spins of e– and which orbital each is in O 1 s 2 s 2 p 3 s 3 p P paired e– unpaired e– valence e–: the s and p e–s (ONLY!) in the outermost energy level
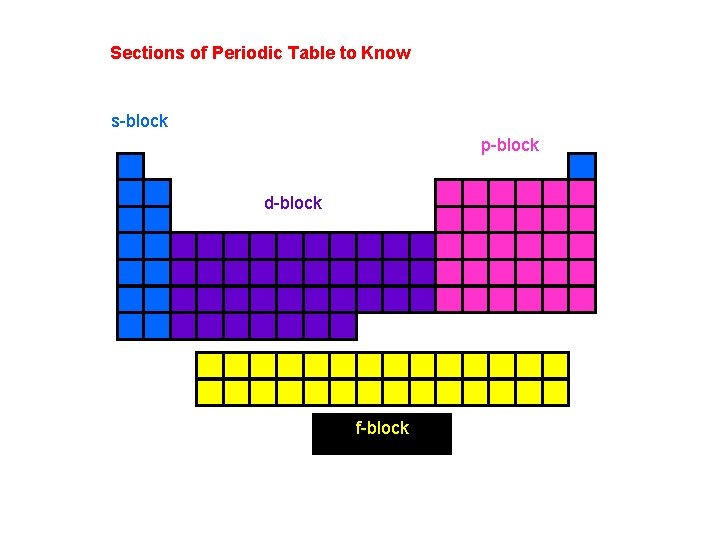
Sections of Periodic Table to Know s-block p-block d-block f-block
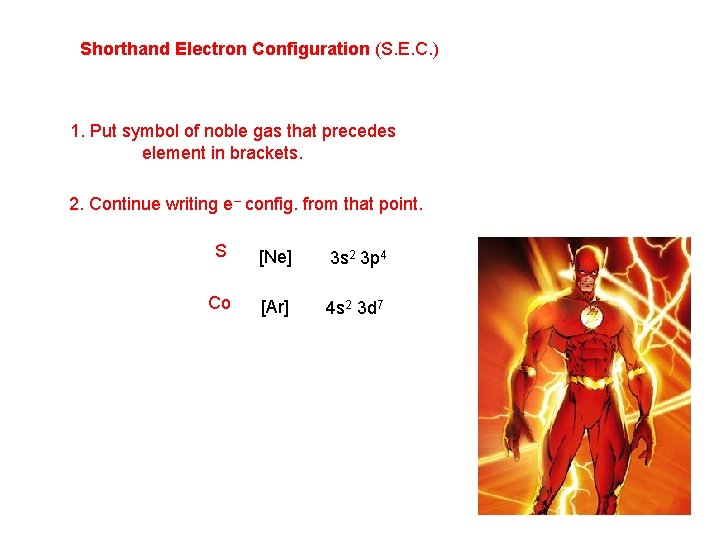
Shorthand Electron Configuration (S. E. C. ) 1. Put symbol of noble gas that precedes element in brackets. 2. Continue writing e– config. from that point. S [Ne] 3 s 2 3 p 4 Co [Ar] 4 s 2 3 d 7
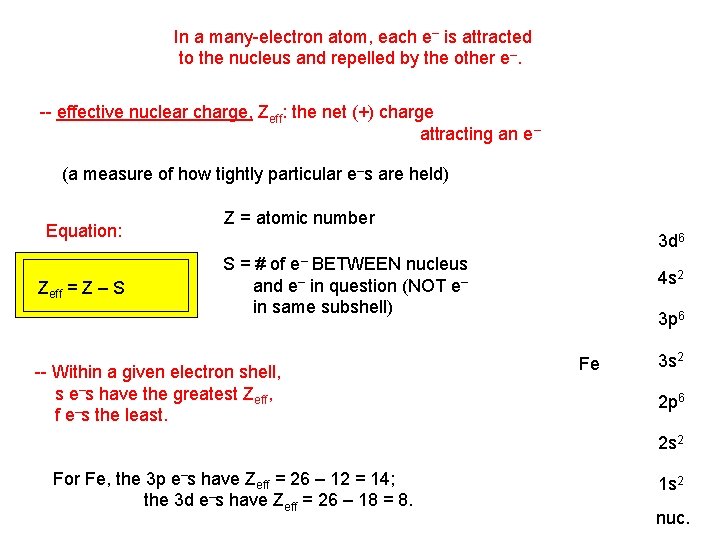
In a many-electron atom, each e– is attracted to the nucleus and repelled by the other e–. -- effective nuclear charge, Zeff: the net (+) charge attracting an e– (a measure of how tightly particular e–s are held) Equation: Zeff = Z – S Z = atomic number 3 d 6 S = # of e– BETWEEN nucleus and e– in question (NOT e– in same subshell) -- Within a given electron shell, s e–s have the greatest Zeff, f e–s the least. 4 s 2 3 p 6 Fe 3 s 2 2 p 6 2 s 2 For Fe, the 3 p e–s have Zeff = 26 – 12 = 14; the 3 d e–s have Zeff = 26 – 18 = 8. 1 s 2 nuc.
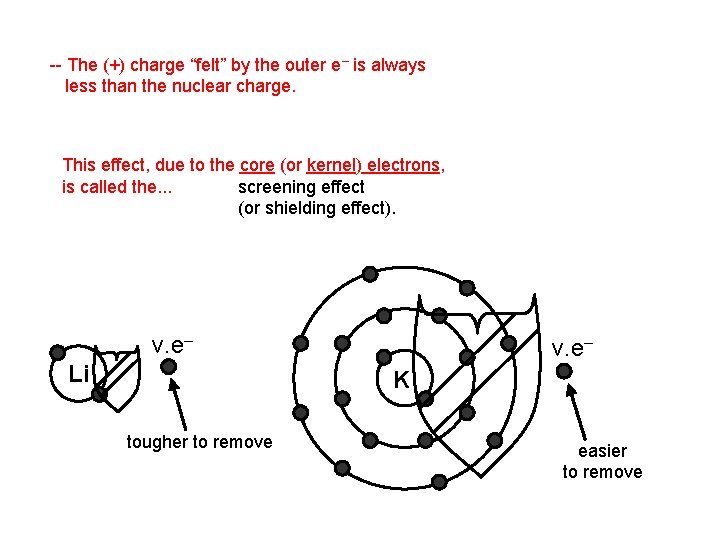
-- The (+) charge “felt” by the outer e– is always less than the nuclear charge. This effect, due to the core (or kernel) electrons, is called the. . . screening effect (or shielding effect). v. e– Li v. e– K tougher to remove easier to remove
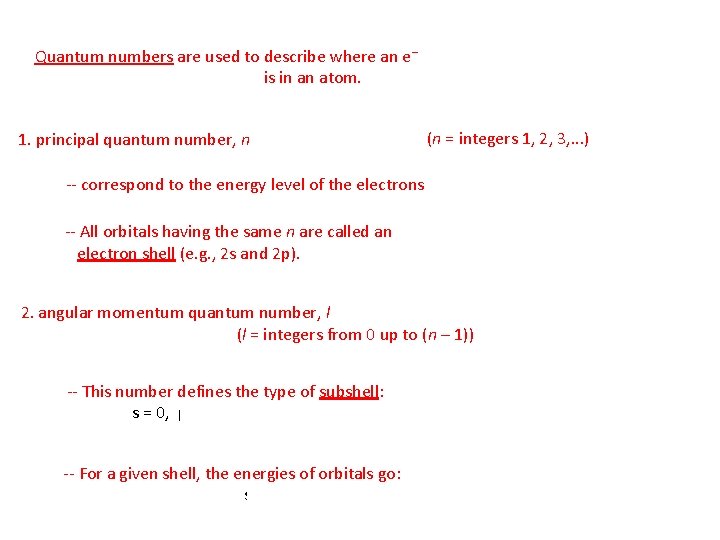
Quantum numbers are used to describe where an e– is in an atom. 1. principal quantum number, n (n = integers 1, 2, 3, . . . ) -- correspond to the energy level of the electrons -- All orbitals having the same n are called an electron shell (e. g. , 2 s and 2 p). 2. angular momentum quantum number, l (l = integers from 0 up to (n – 1)) -- This number defines the type of subshell: s = 0, p = 1, d = 2, f = 3 -- For a given shell, the energies of orbitals go: s<p<d<f
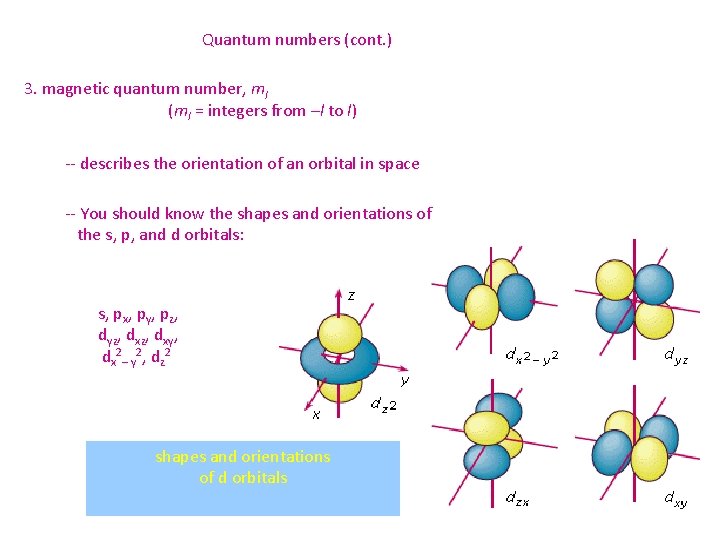
Quantum numbers (cont. ) 3. magnetic quantum number, ml (ml = integers from –l to l) -- describes the orientation of an orbital in space -- You should know the shapes and orientations of the s, p, and d orbitals: s, px, py, pz, dyz, dxy, dx 2– y 2, dz 2 shapes and orientations of d orbitals
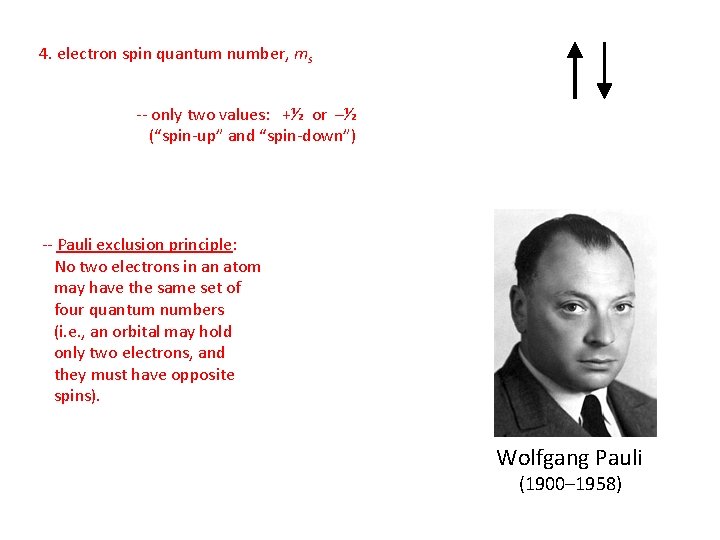
4. electron spin quantum number, ms -- only two values: +½ or –½ (“spin-up” and “spin-down”) -- Pauli exclusion principle: No two electrons in an atom may have the same set of four quantum numbers (i. e. , an orbital may hold only two electrons, and they must have opposite spins). Wolfgang Pauli (1900– 1958)
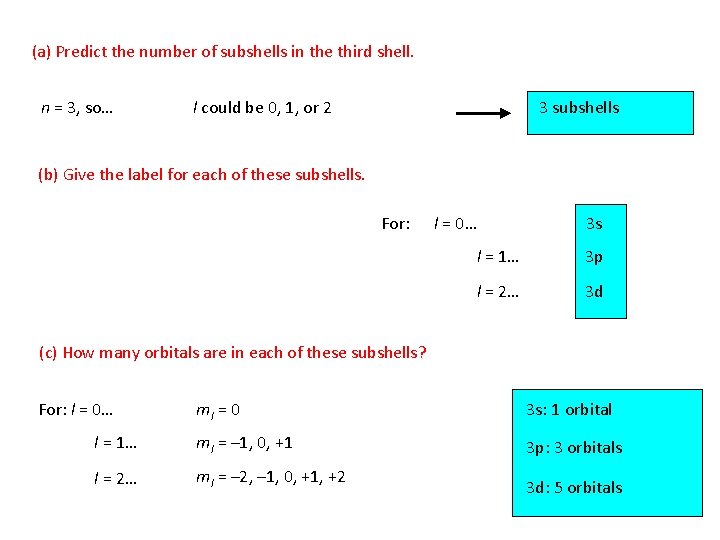
(a) Predict the number of subshells in the third shell. n = 3, so… l could be 0, 1, or 2 3 subshells (b) Give the label for each of these subshells. For: l = 0… 3 s l = 1… 3 p l = 2… 3 d (c) How many orbitals are in each of these subshells? For: l = 0… ml = 0 3 s: 1 orbital l = 1… ml = – 1, 0, +1 3 p: 3 orbitals l = 2… ml = – 2, – 1, 0, +1, +2 3 d: 5 orbitals
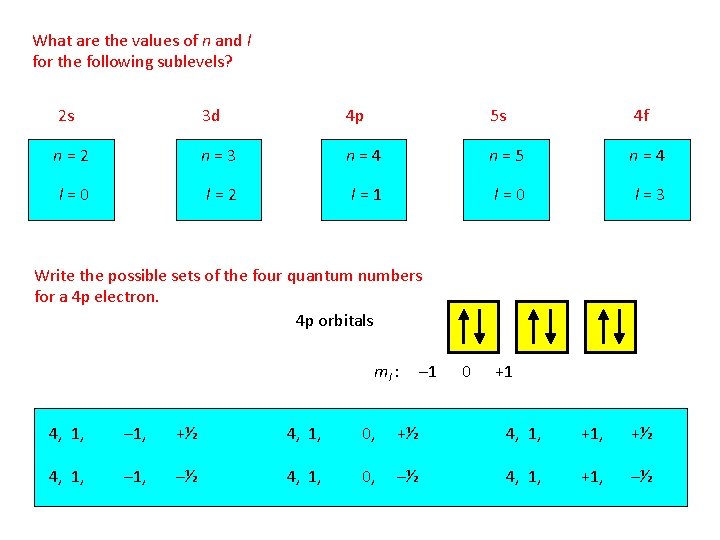
What are the values of n and l for the following sublevels? 2 s 3 d 4 p 5 s 4 f n=2 n=3 n=4 n=5 n=4 l=0 l=2 l=1 l=0 l=3 Write the possible sets of the four quantum numbers for a 4 p electron. 4 p orbitals ml : – 1 0 +1 4, 1, – 1, +½ 4, 1, 0, +½ 4, 1, –½ 4, 1, 0, –½ 4, 1, +1, –½
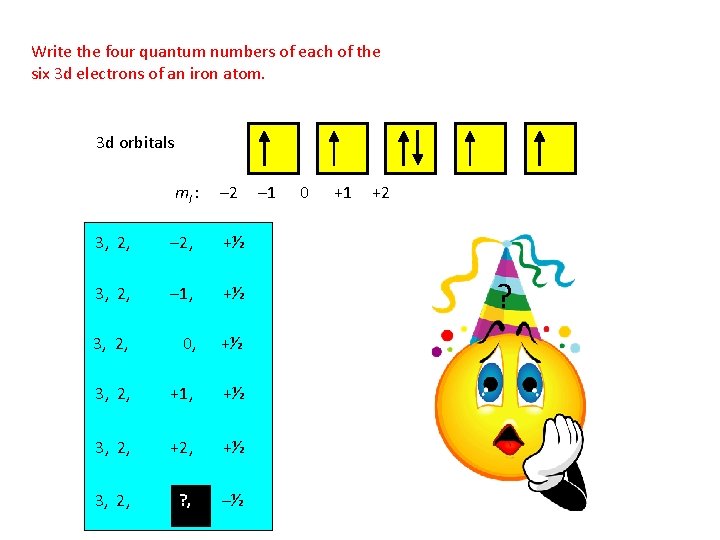
Write the four quantum numbers of each of the six 3 d electrons of an iron atom. 3 d orbitals ml : – 2 3, 2, – 2, +½ 3, 2, – 1, +½ 3, 2, 0, +½ 3, 2, +1, +½ 3, 2, ? , –½ – 1 0 +1 +2 ?
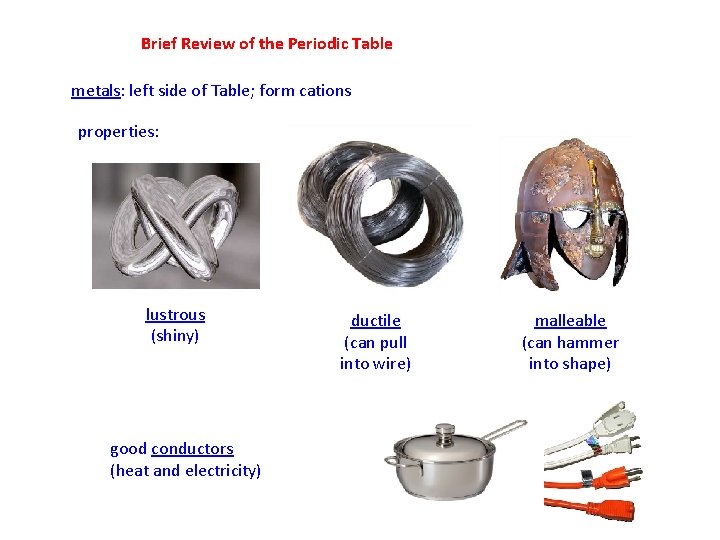
Brief Review of the Periodic Table metals: left side of Table; form cations properties: lustrous (shiny) good conductors (heat and electricity) ductile (can pull into wire) malleable (can hammer into shape)
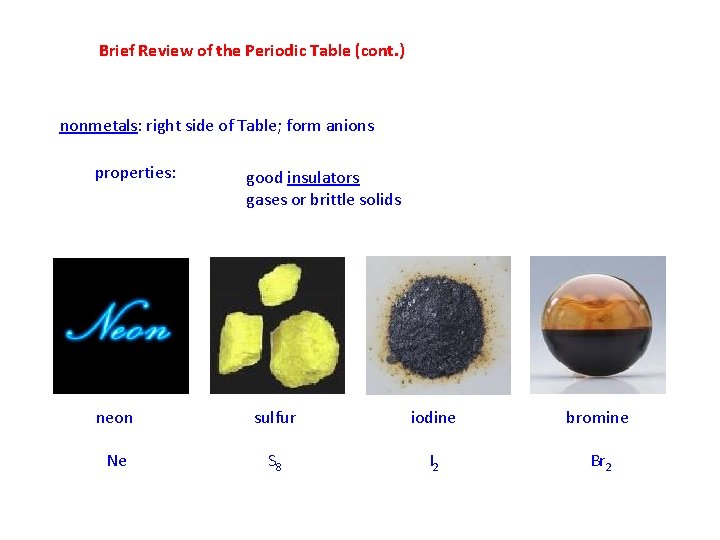
Brief Review of the Periodic Table (cont. ) nonmetals: right side of Table; form anions properties: good insulators gases or brittle solids neon sulfur iodine bromine Ne S 8 I 2 Br 2
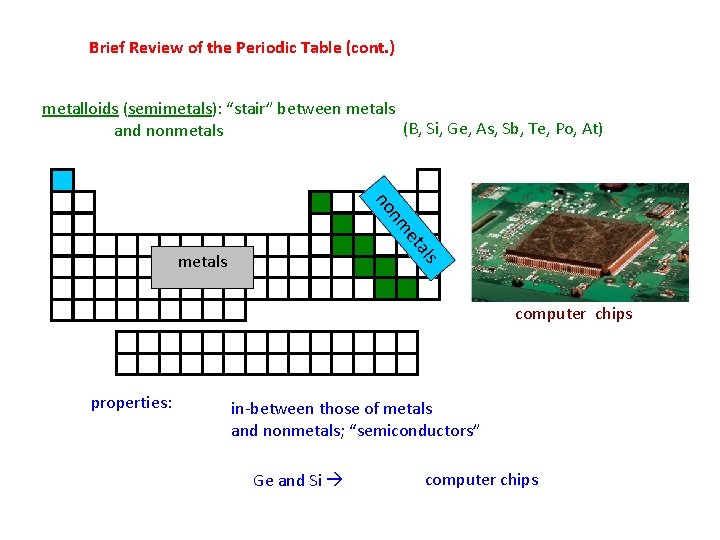
Brief Review of the Periodic Table (cont. ) metalloids (semimetals): “stair” between metals (B, Si, Ge, As, Sb, Te, Po, At) and nonmetals et nm no Si and Ge metals computer chips properties: in-between those of metals and nonmetals; “semiconductors” Ge and Si computer chips
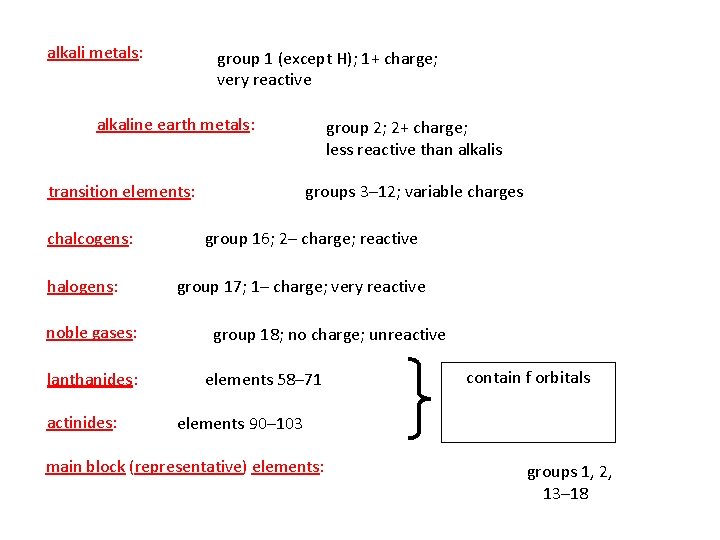
alkali metals: group 1 (except H); 1+ charge; very reactive alkaline earth metals: groups 3– 12; variable charges transition elements: chalcogens: halogens: noble gases: lanthanides: actinides: group 2; 2+ charge; less reactive than alkalis group 16; 2– charge; reactive group 17; 1– charge; very reactive group 18; no charge; unreactive elements 58– 71 contain f orbitals elements 90– 103 main block (representative) elements: groups 1, 2, 13– 18
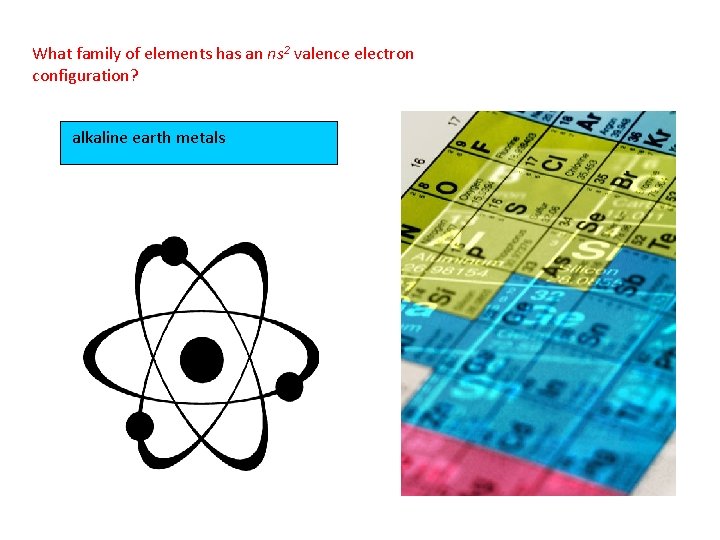
What family of elements has an ns 2 valence electron configuration? alkaline earth metals
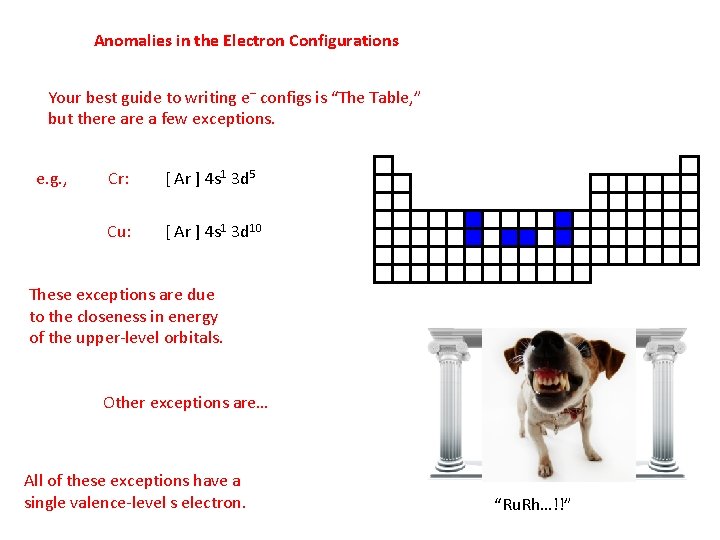
Anomalies in the Electron Configurations Your best guide to writing e– configs is “The Table, ” but there a few exceptions. e. g. , Cr: [ Ar ] 4 s 1 3 d 5 Cu: [ Ar ] 4 s 1 3 d 10 These exceptions are due to the closeness in energy of the upper-level orbitals. Other exceptions are… Mo, Ru, Rh, and Ag. All of these exceptions have a single valence-level s electron. “Ru. Rh…!!”
Ap chemistry electronic structure of atoms
Chapter 6 electronic structure of atoms answers
Quantum theory and the electronic structure of atoms
Which of the d orbitals most resembles a pz orbital?
Regents periodic table
Is the electronic exchange of money or scrip
Electronic field production examples
Electron configuration rule
Ib organic chemistry functional groups
Inorganic vs organic chemistry
Chapter 4 section 2 the structure of atoms answer key
Group 7 halogens
Pn junction band diagram
Atomic structure and properties ap chemistry
Ib chemistry atomic structure
Ap chemistry atomic structure and periodicity
Oxygen periodic trends
Lewis dot structure ch4
First ionization energy of calcium equation
Atoms want
What is expanded octet
What is the relationship between atoms and elements
What are atoms?
Kesler science answer key
The atoms family atomic math challenge answer key
Ionic nonpolar covalent polar covalent
How to count atoms in a formula
Atoms in increasing size
Atoms as the smallest building block of matter
Atom family song
Whats nucleus
Electrons in atoms section 1 light and quantized energy
Atoms with 4 valence electrons
Diamagnetic elements
Periodic table reference table
Grams to moles
16:00
Metallic bonding occurs between
Atoms escape room digital locks answers
Mri hydrogen atoms