Section A Applied Anatomy and Physiology 11 Structure
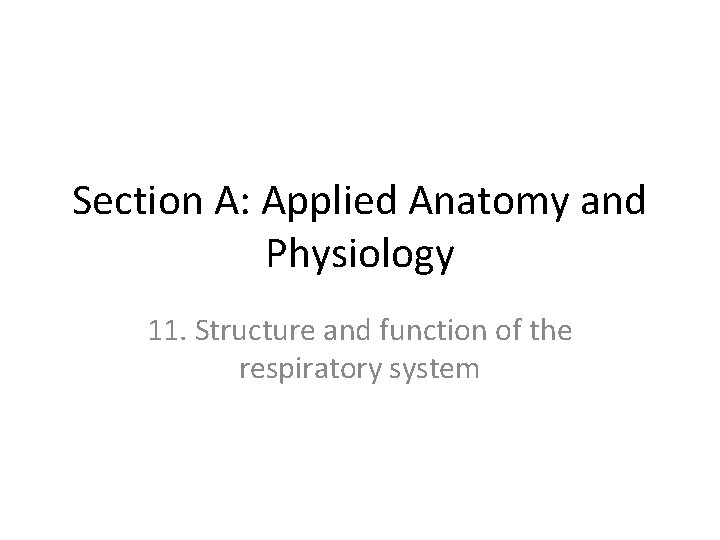
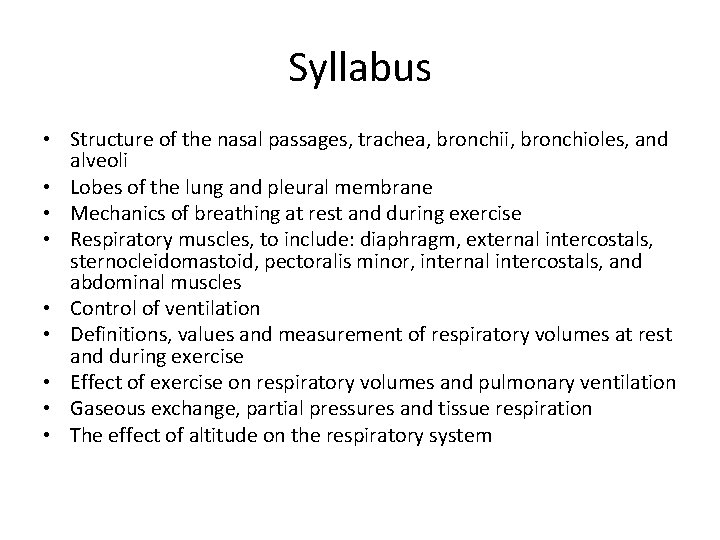
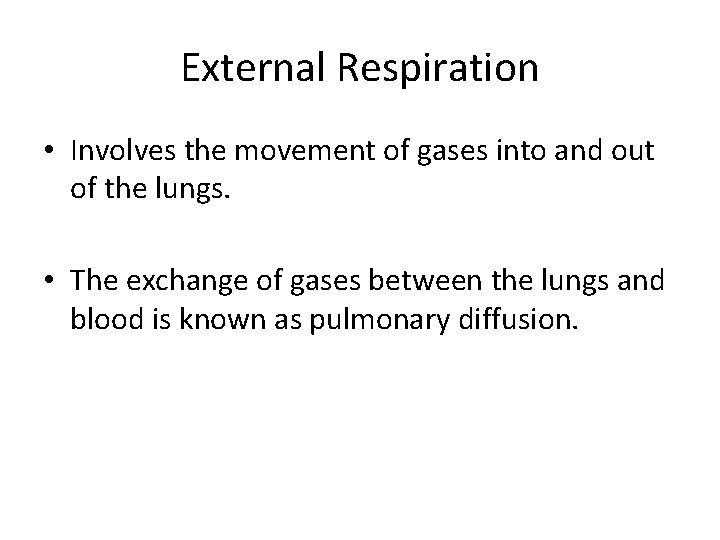
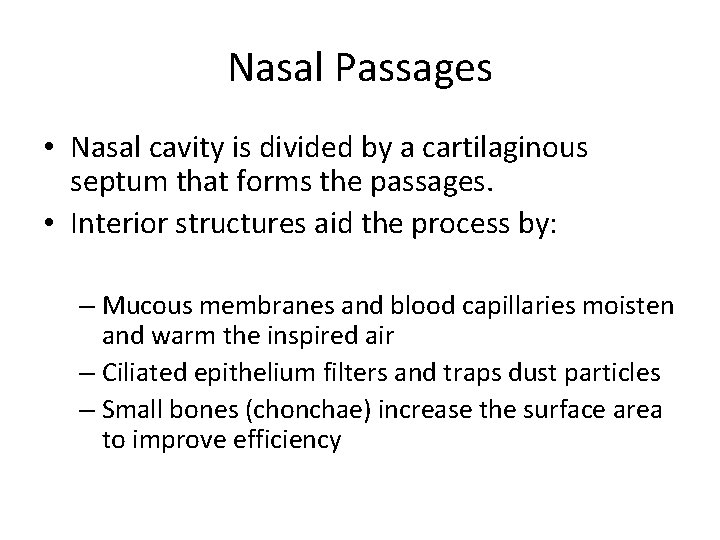
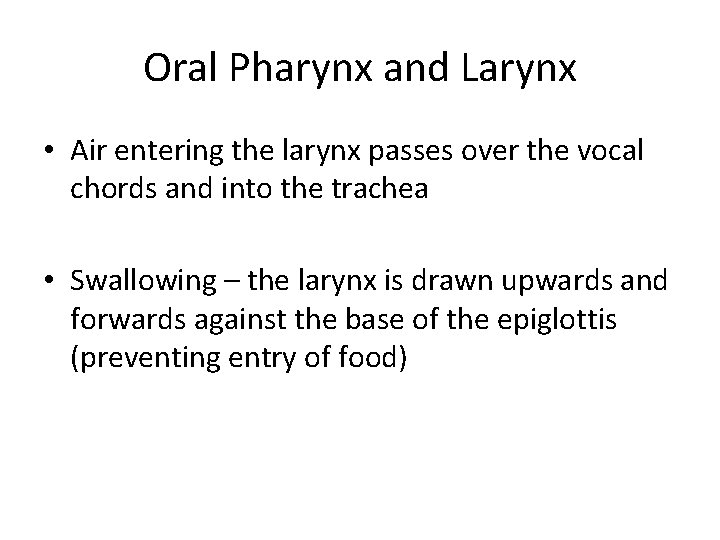
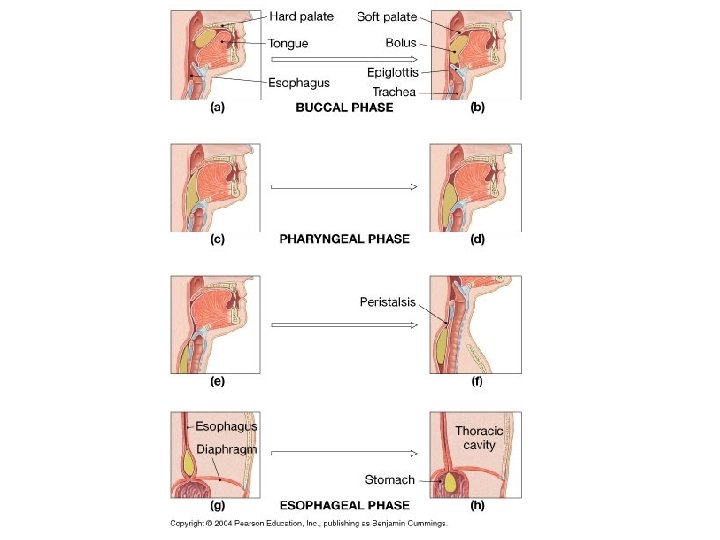
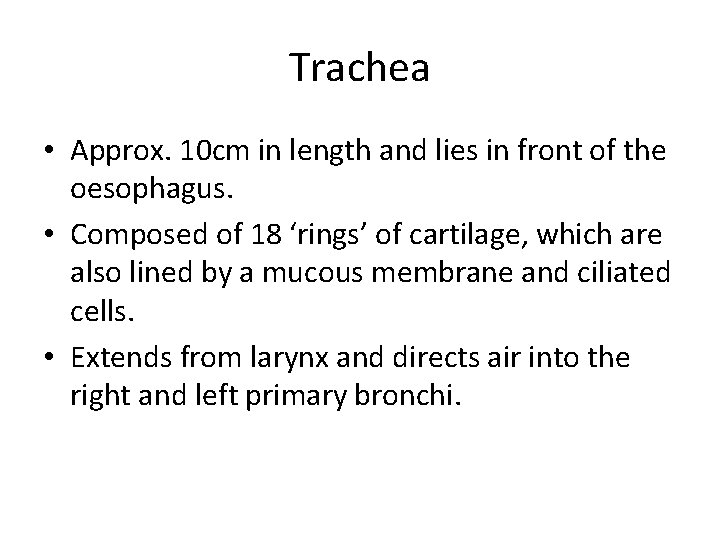
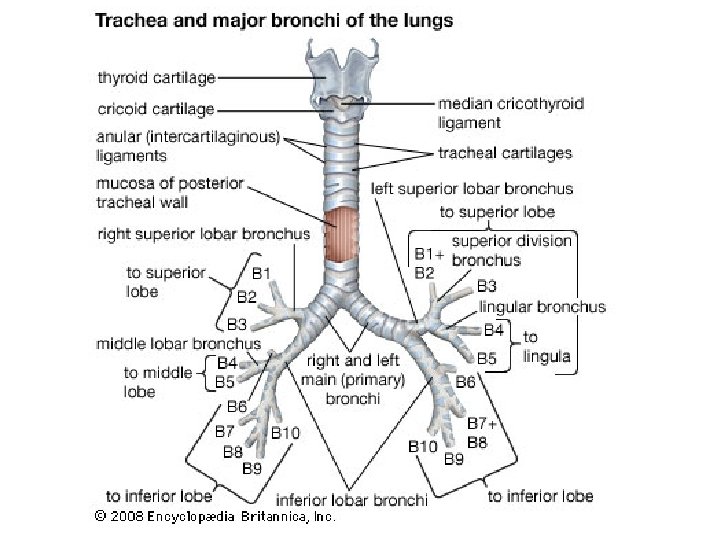
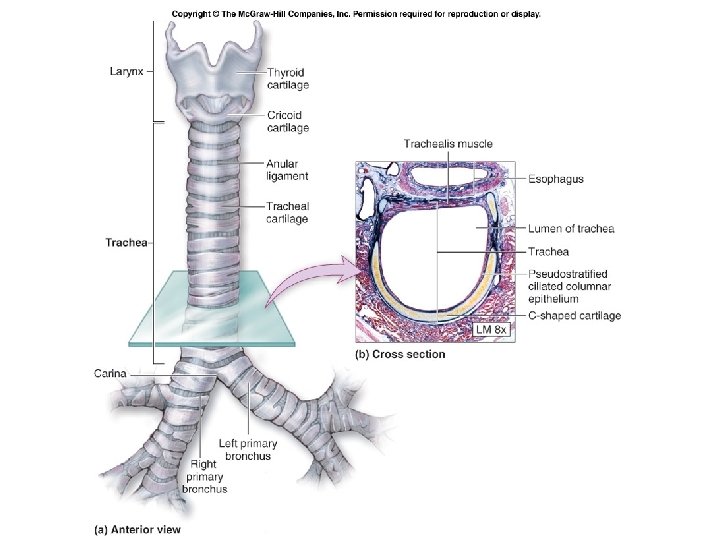
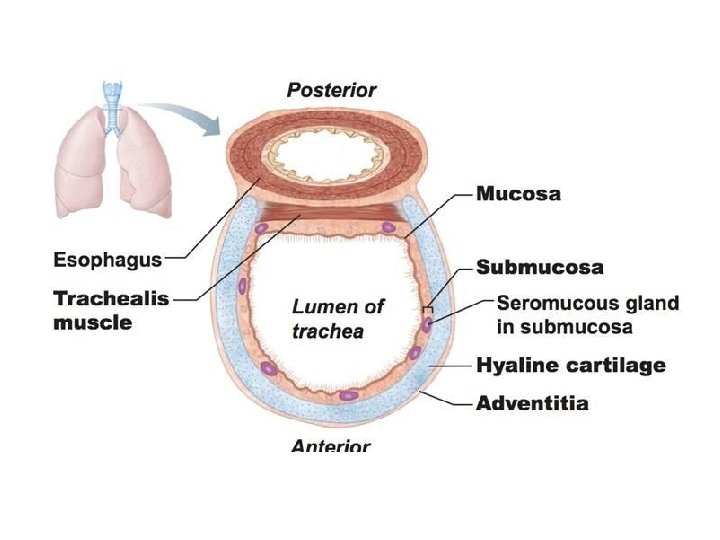
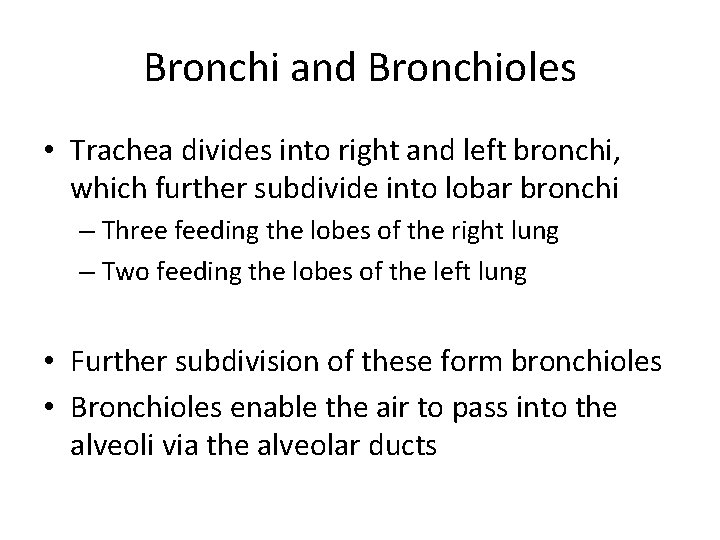
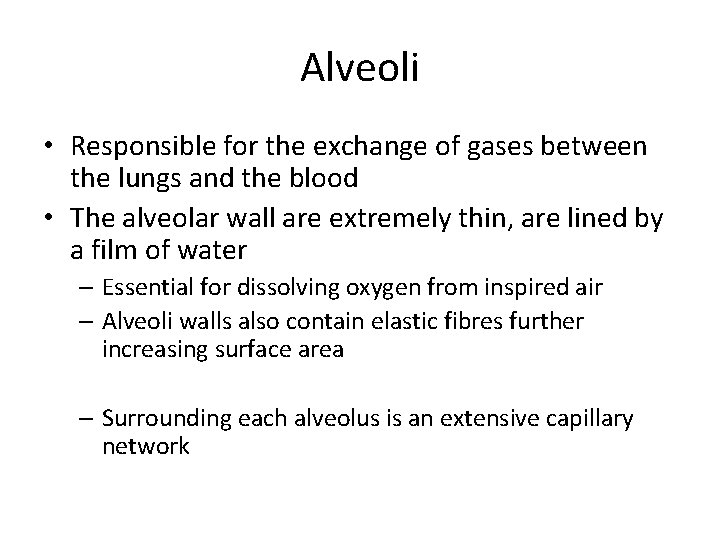
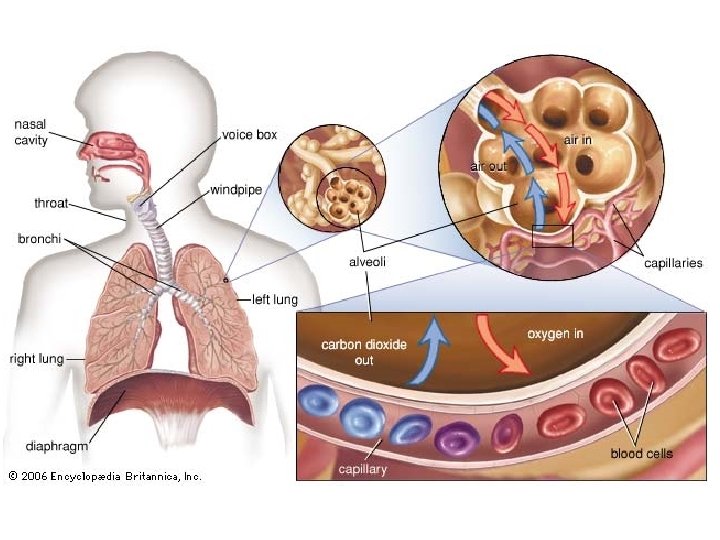
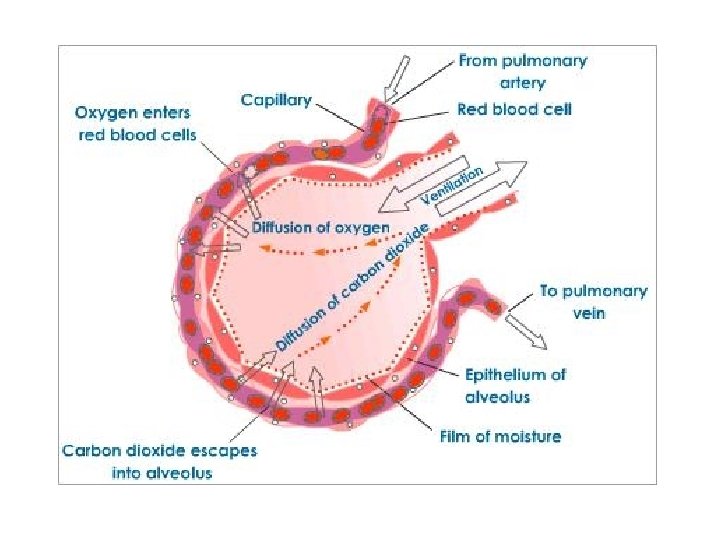
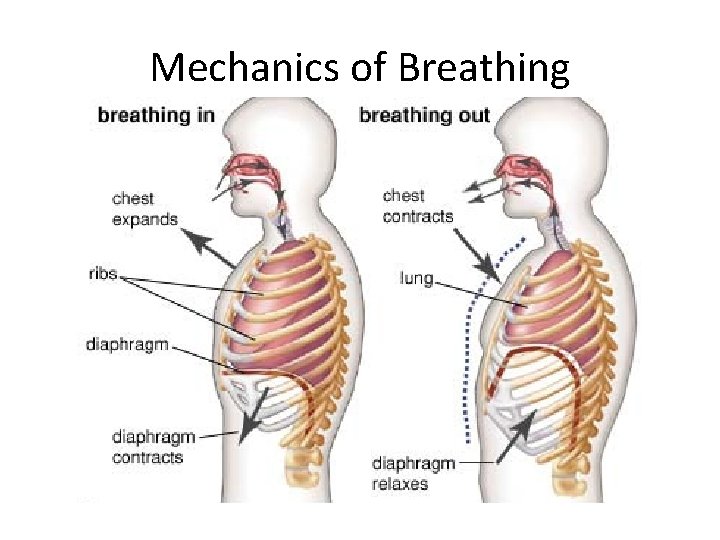
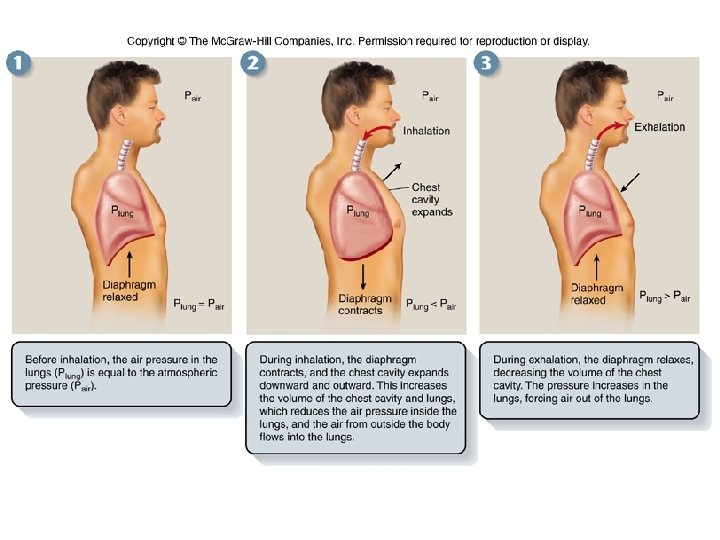
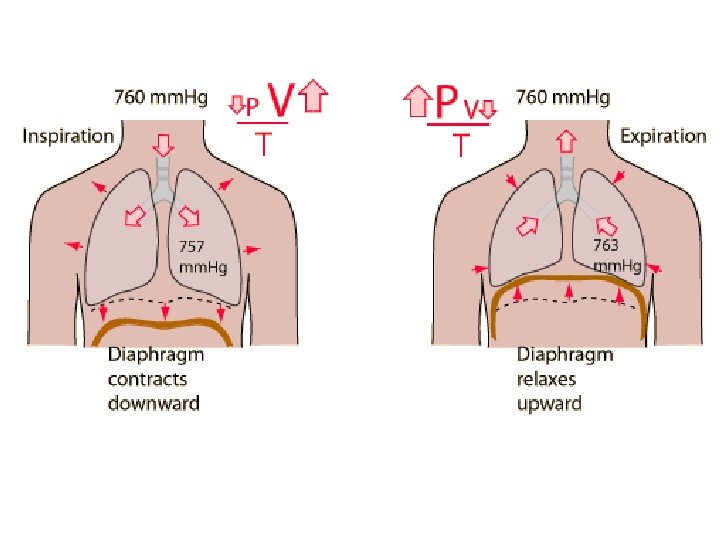
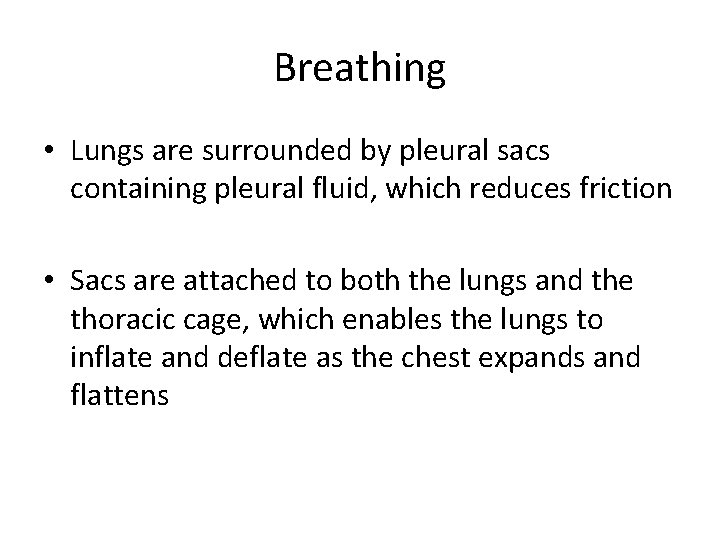
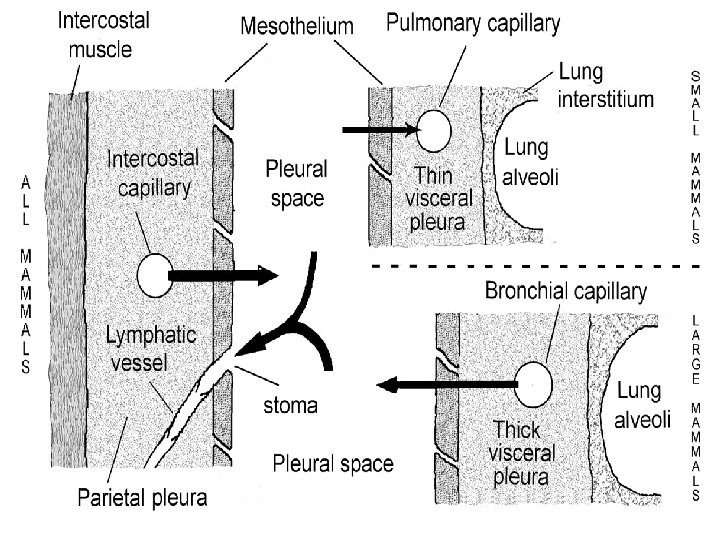
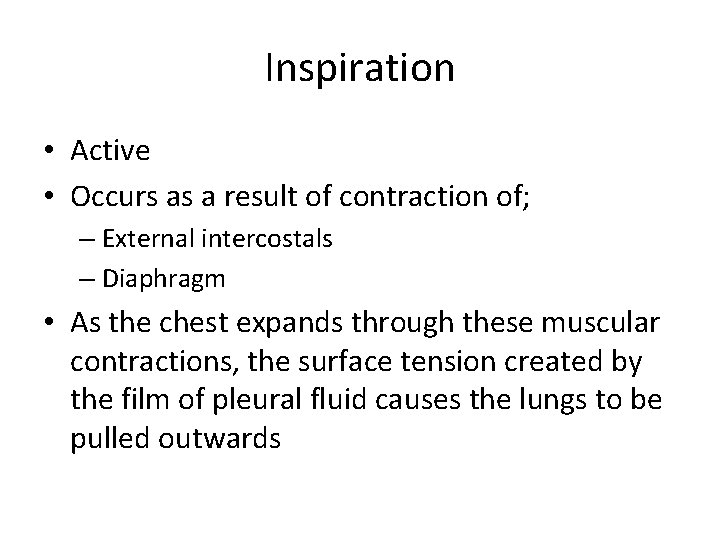
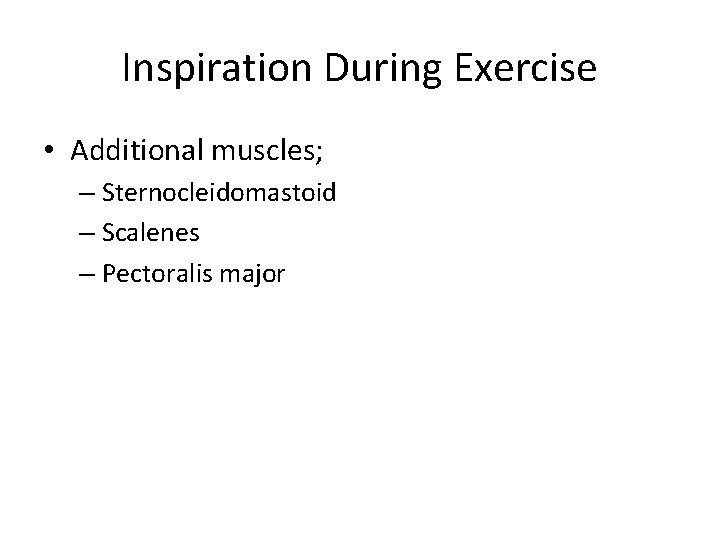
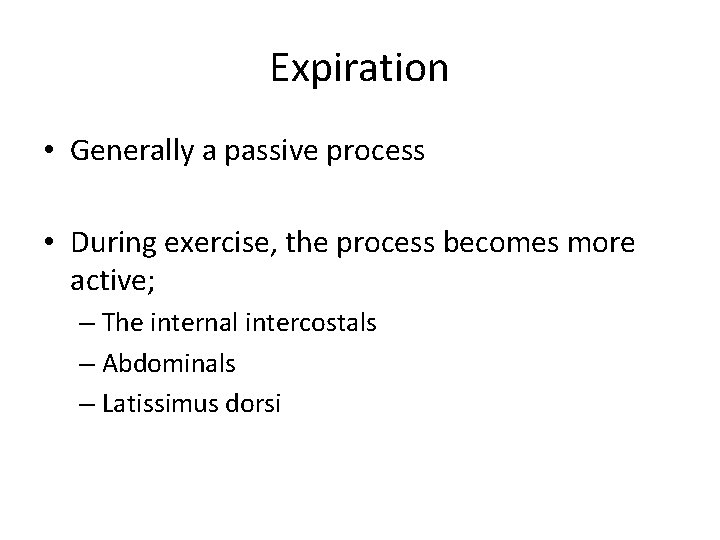
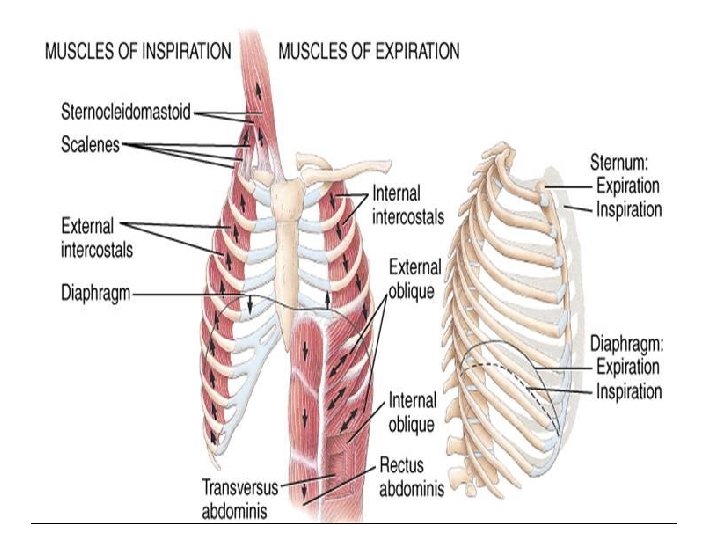
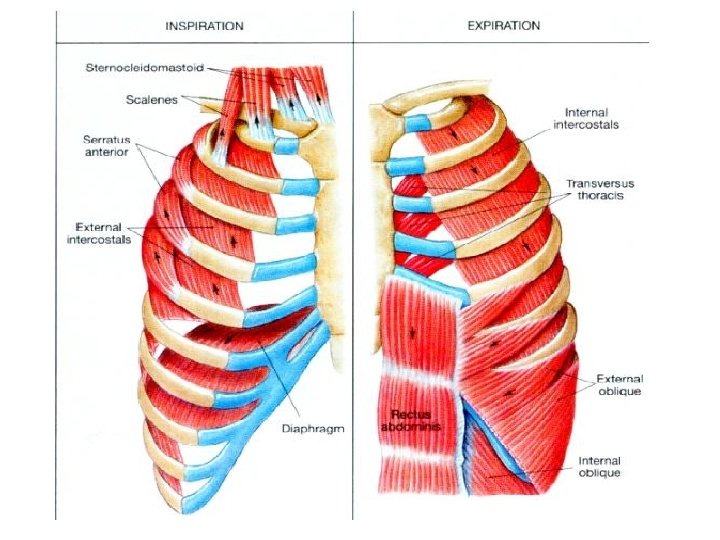
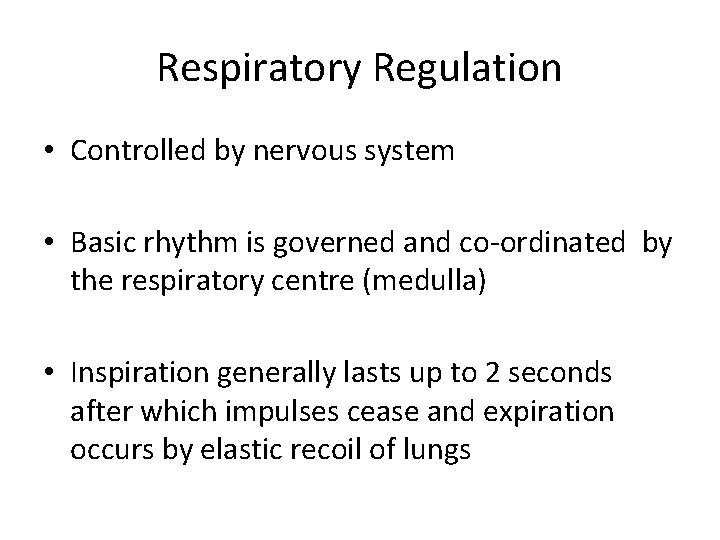
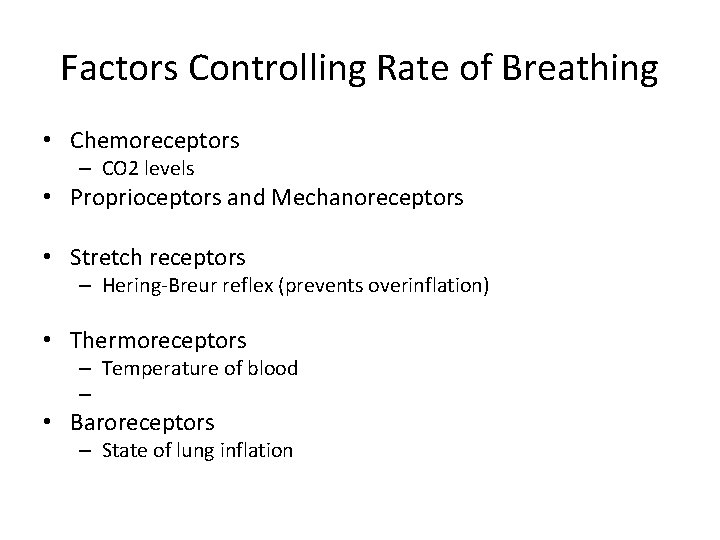
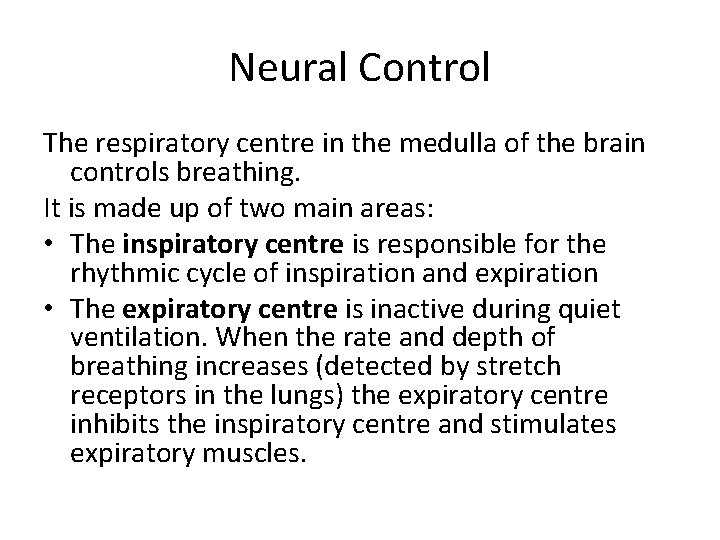
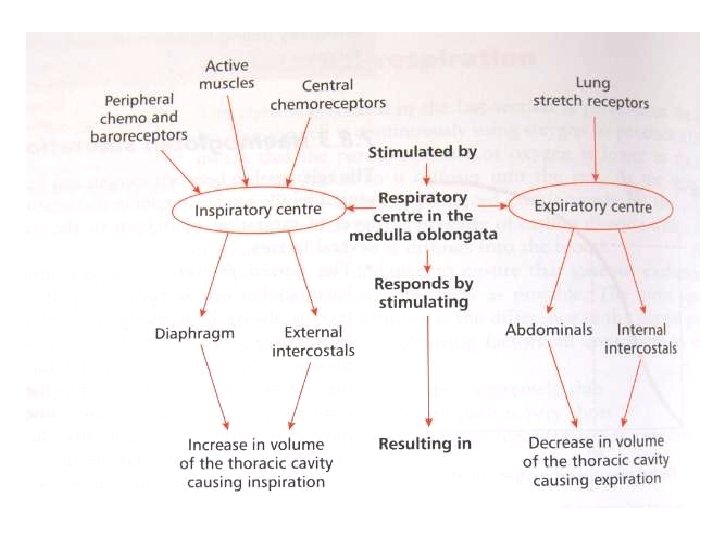
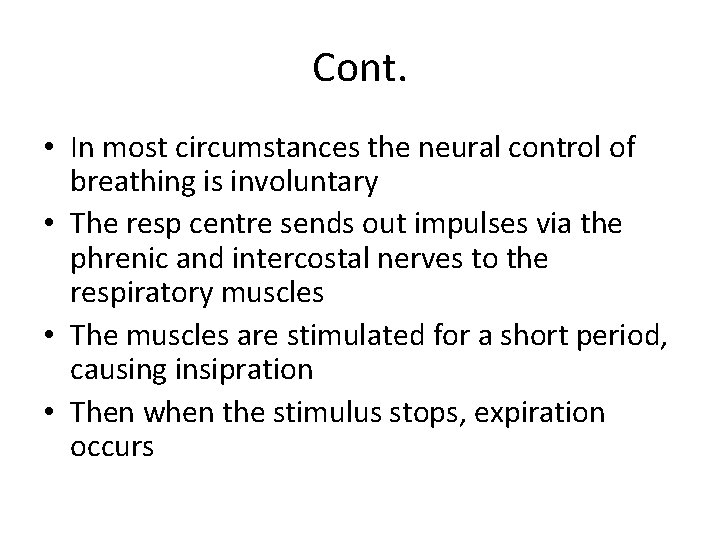
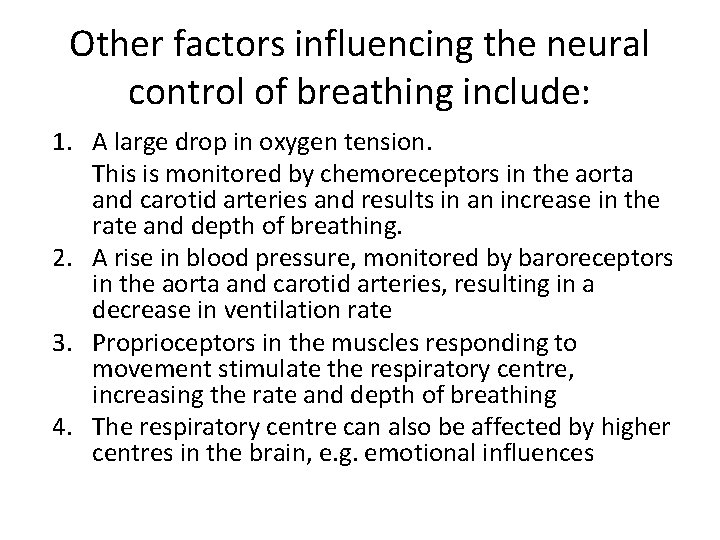
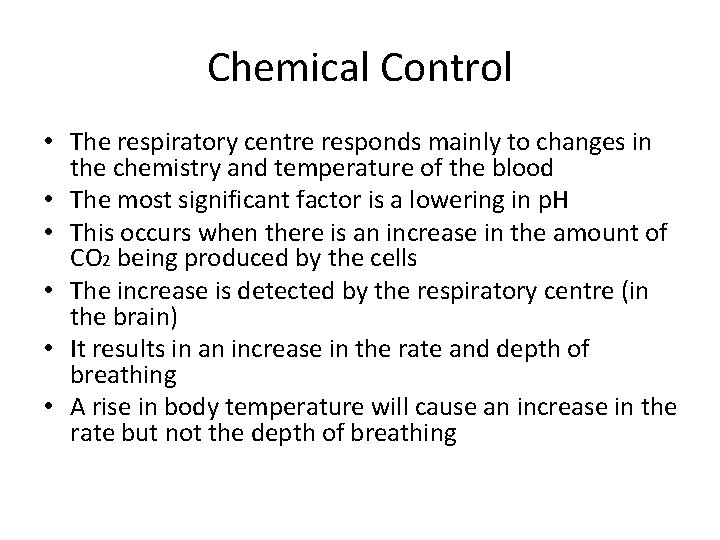
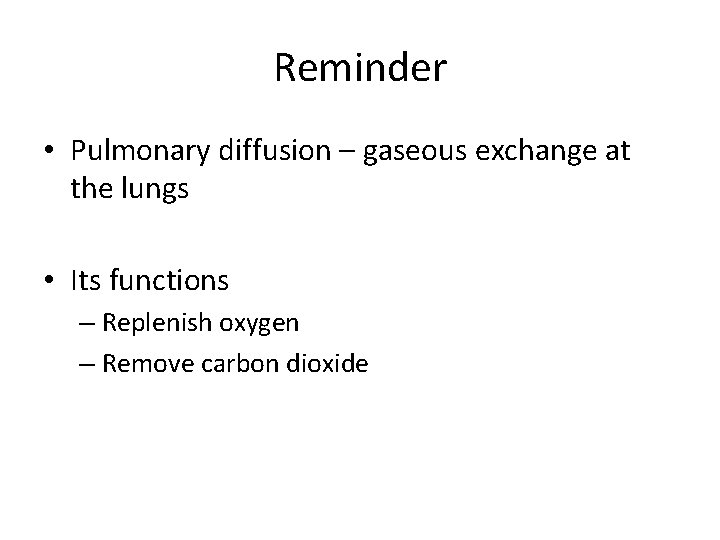
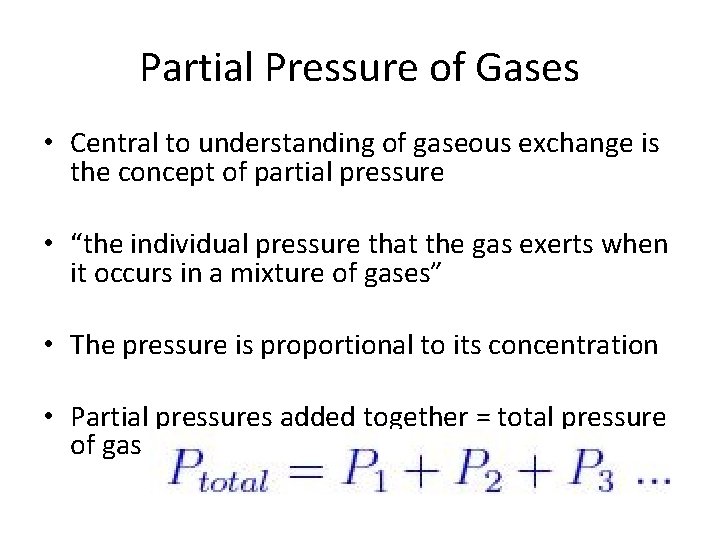
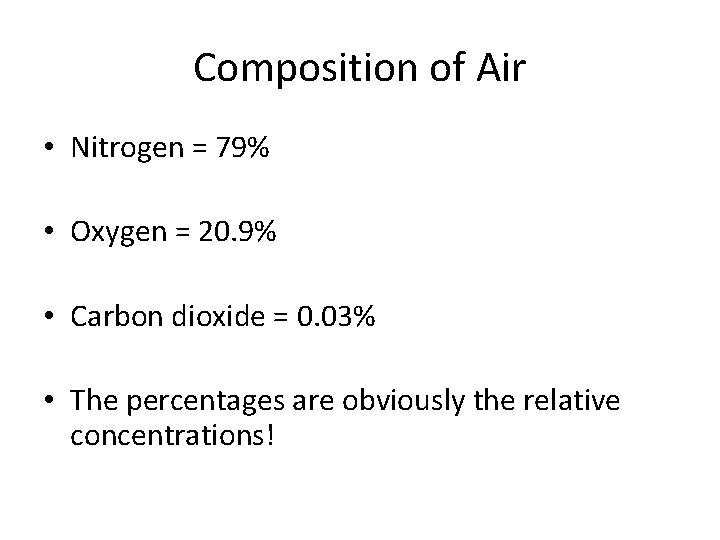
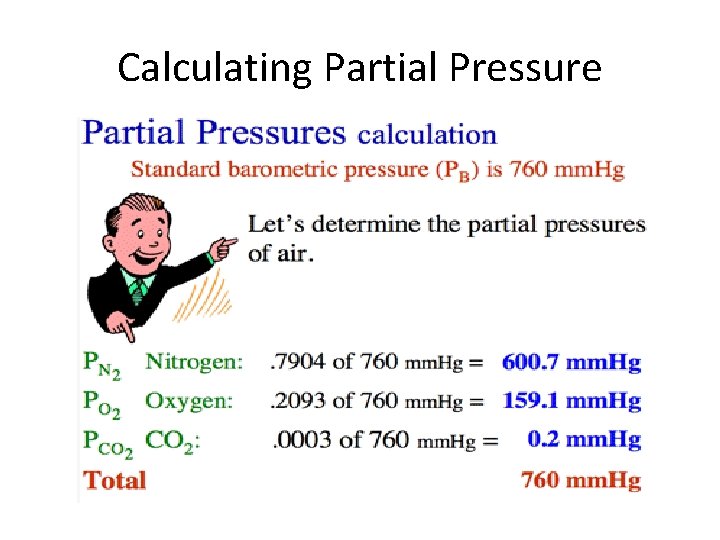
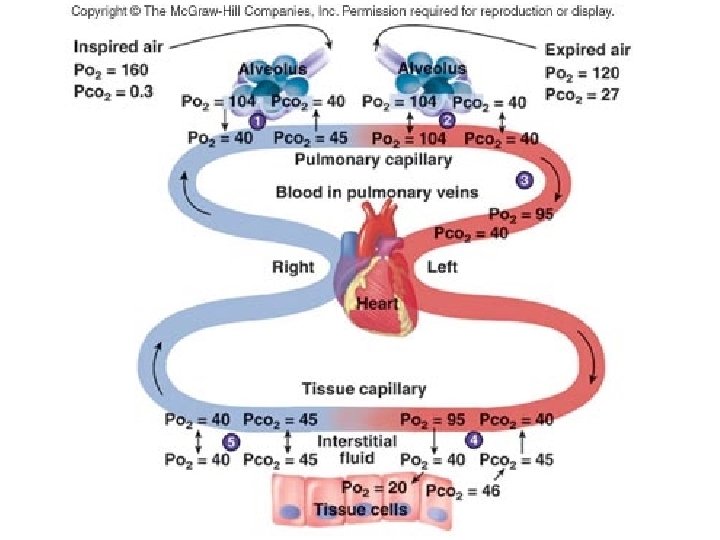
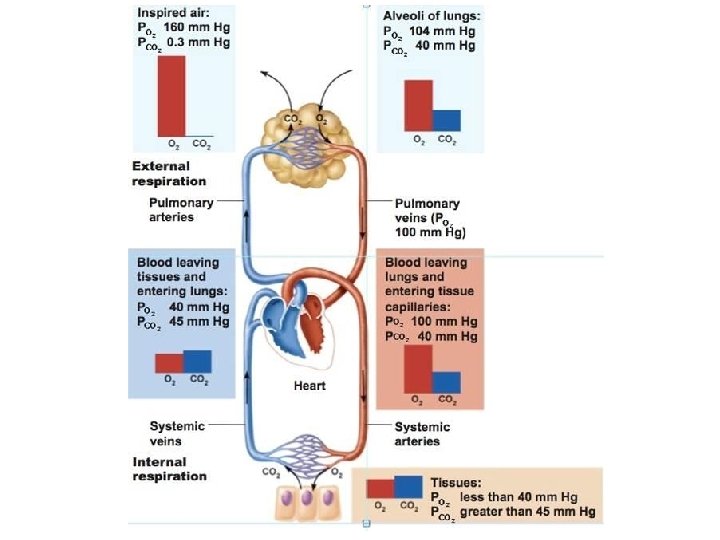
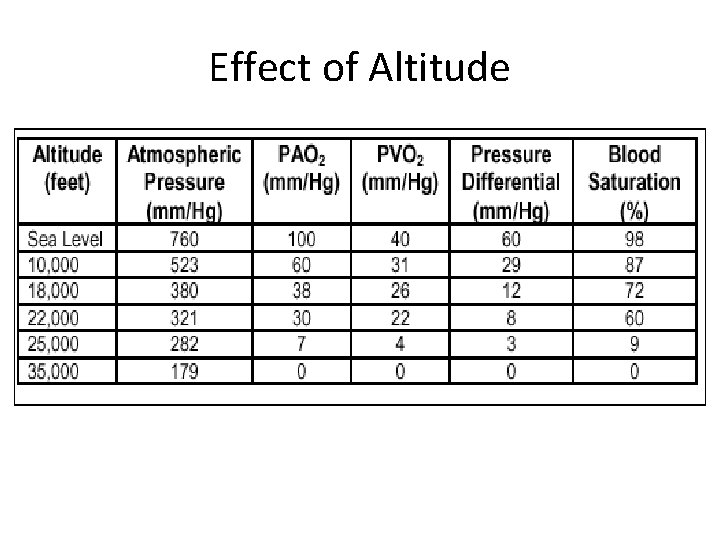
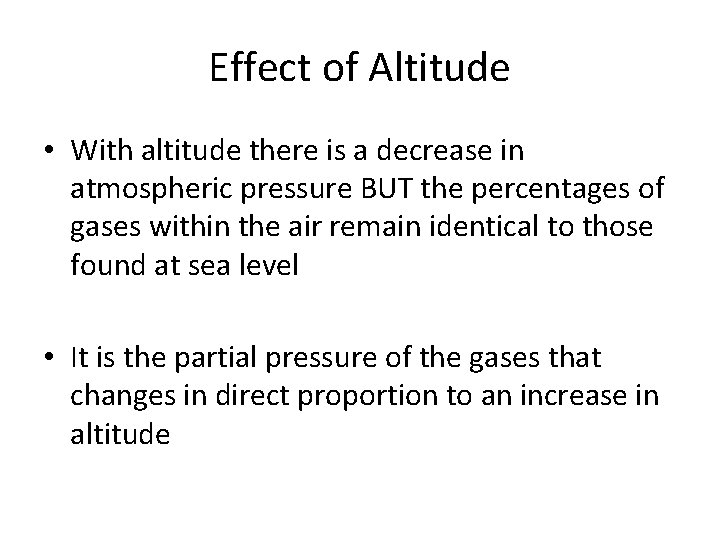
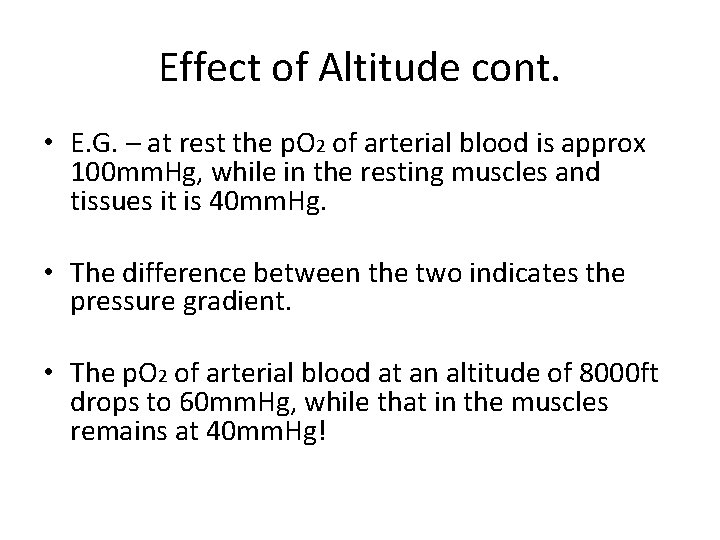
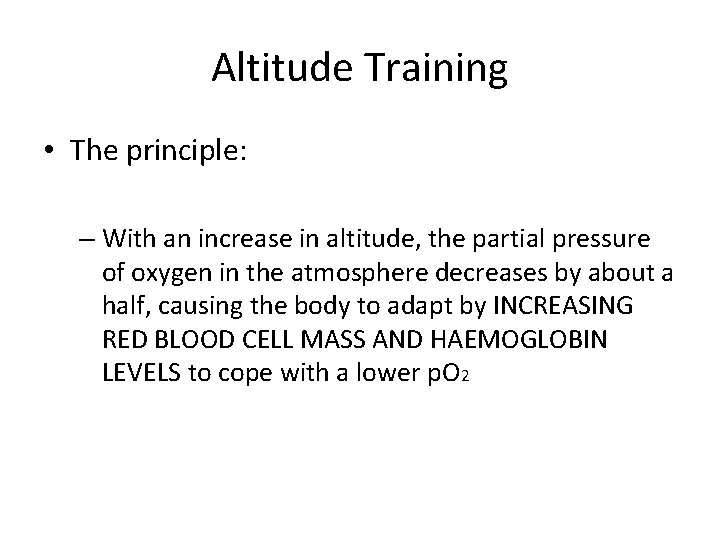
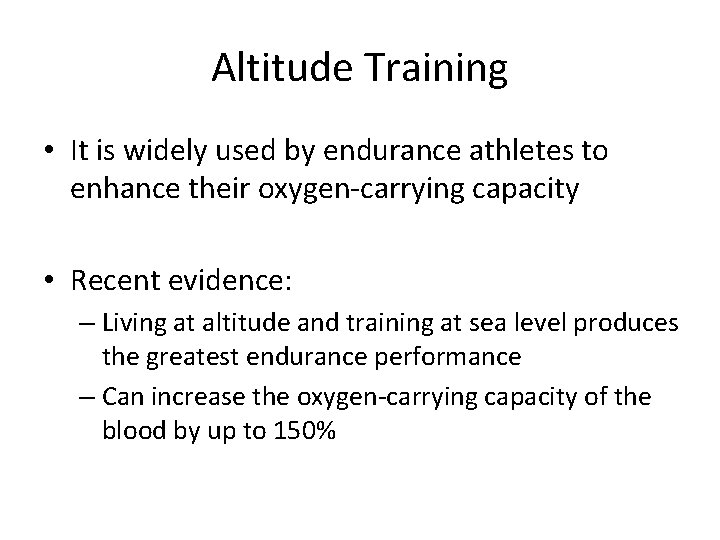
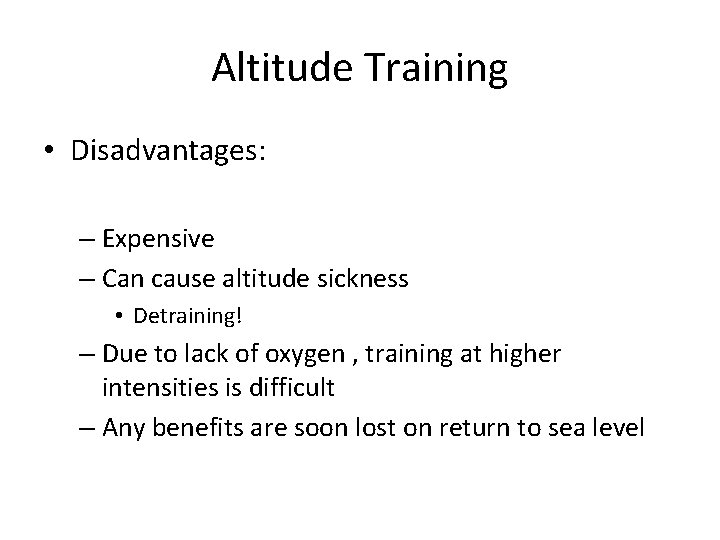
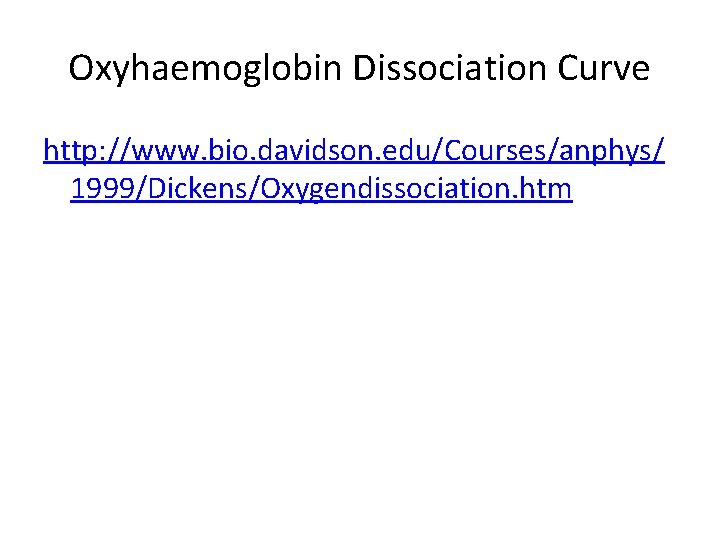
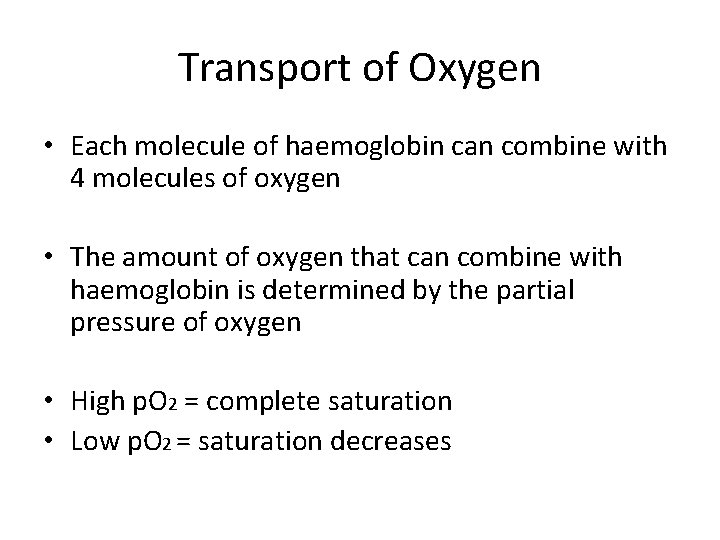
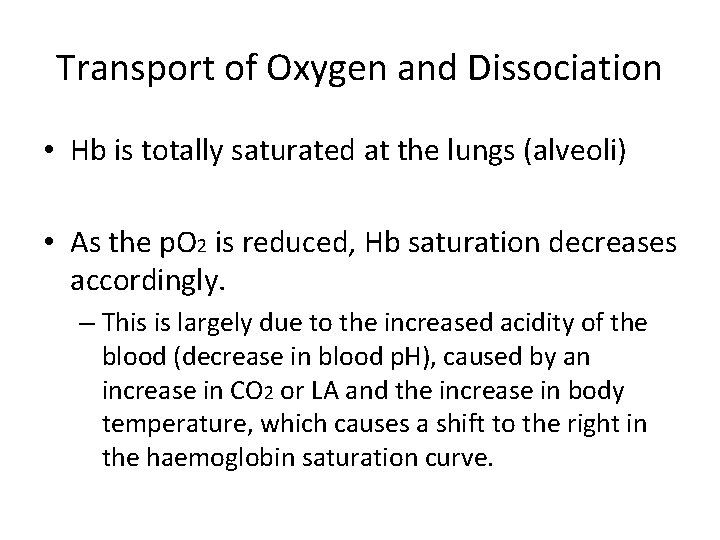
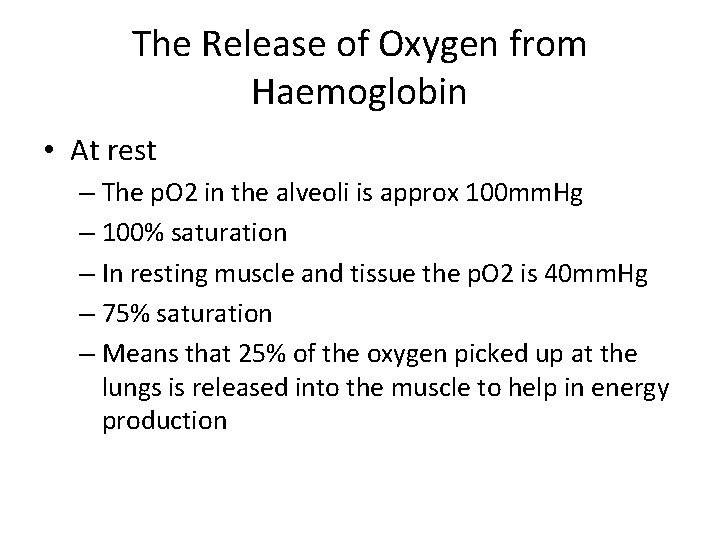
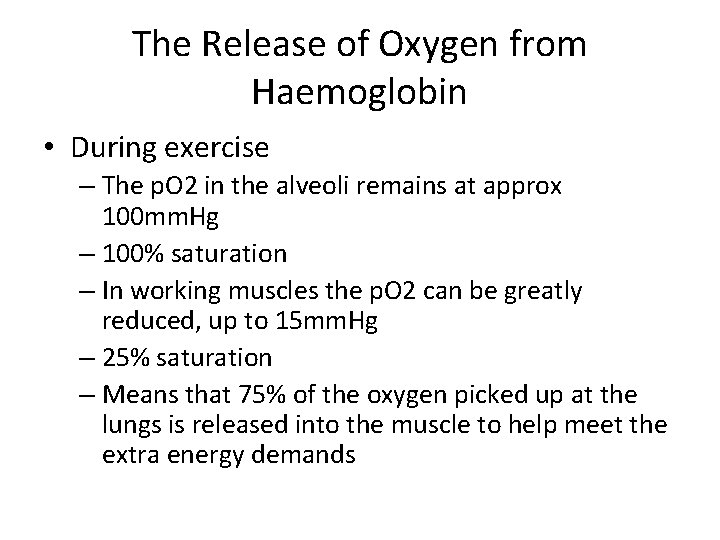
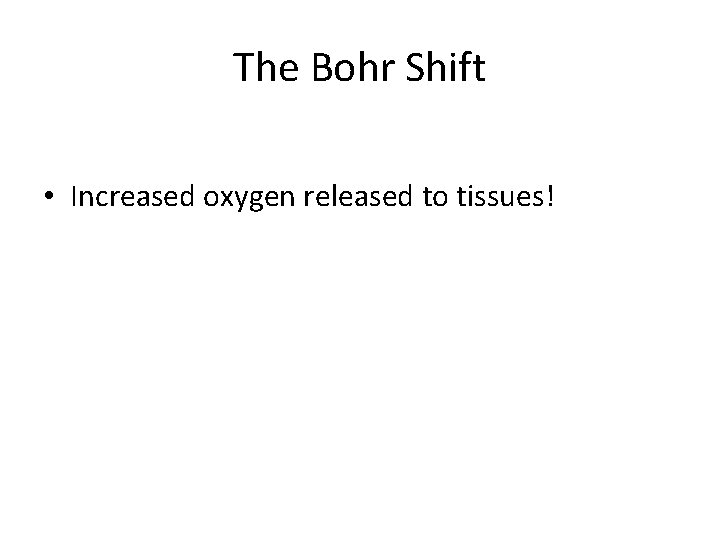
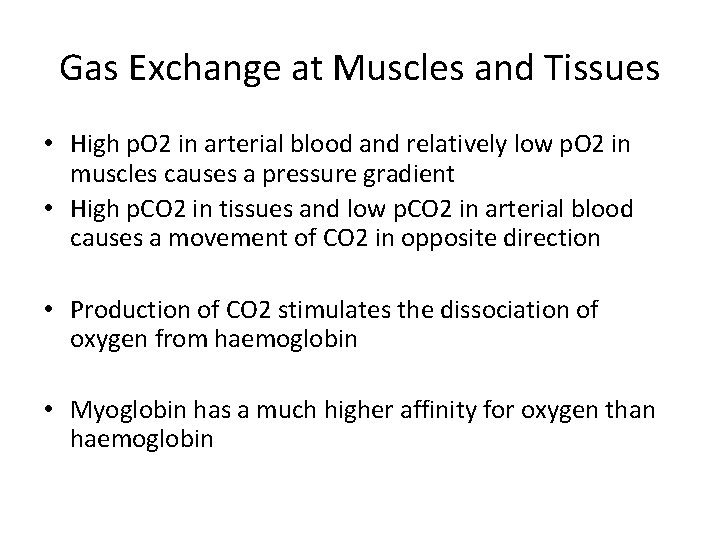
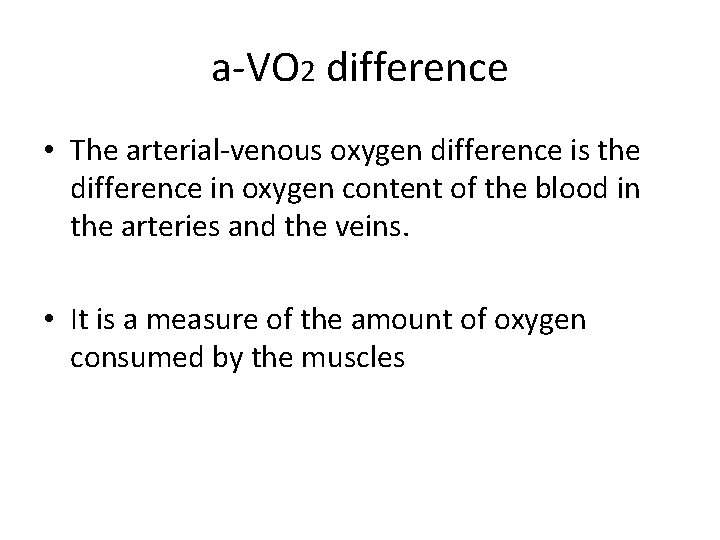
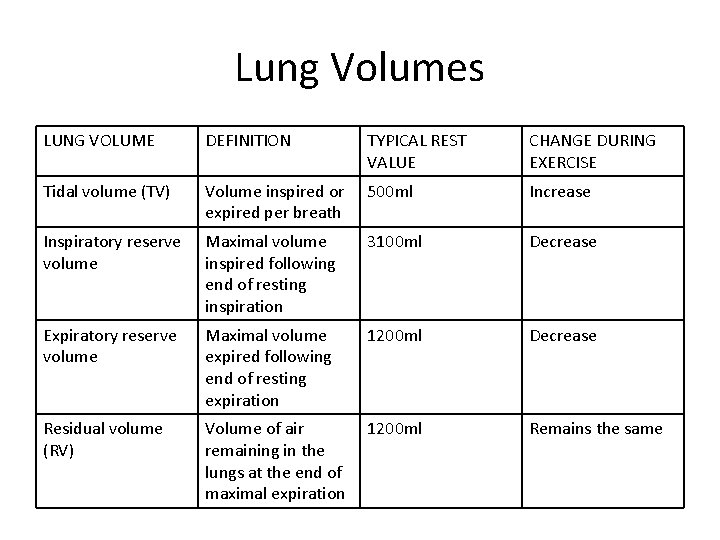
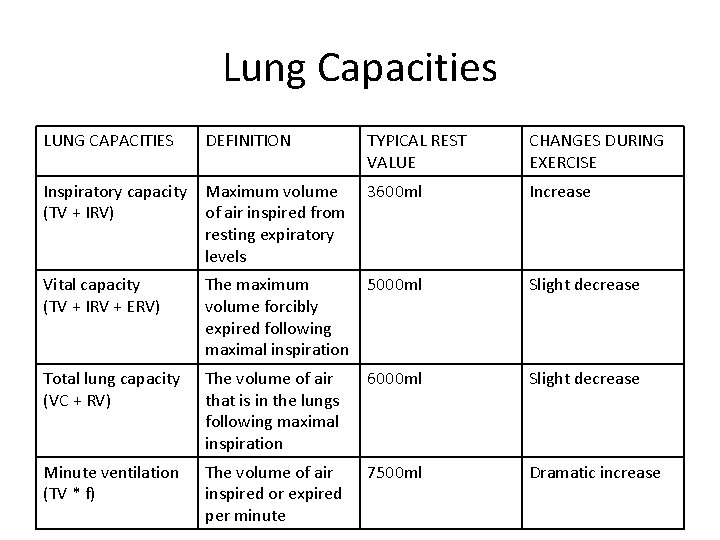
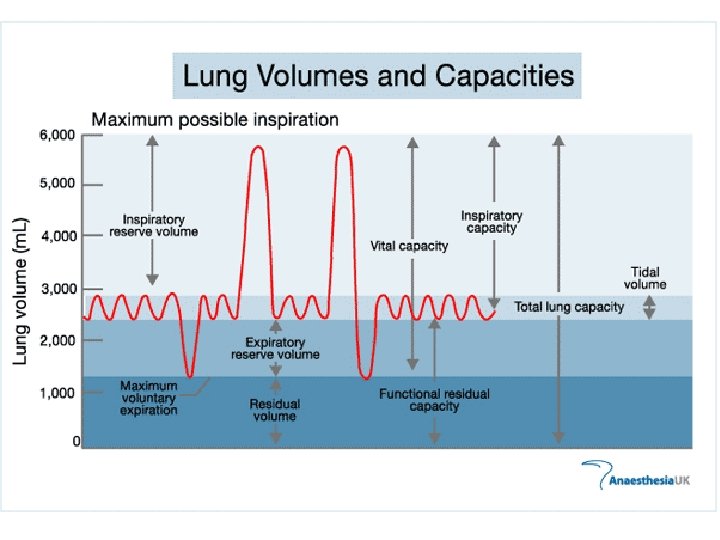
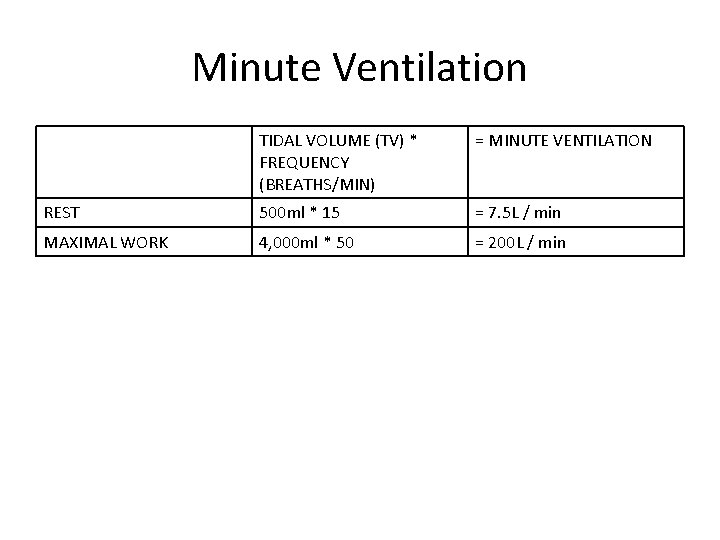
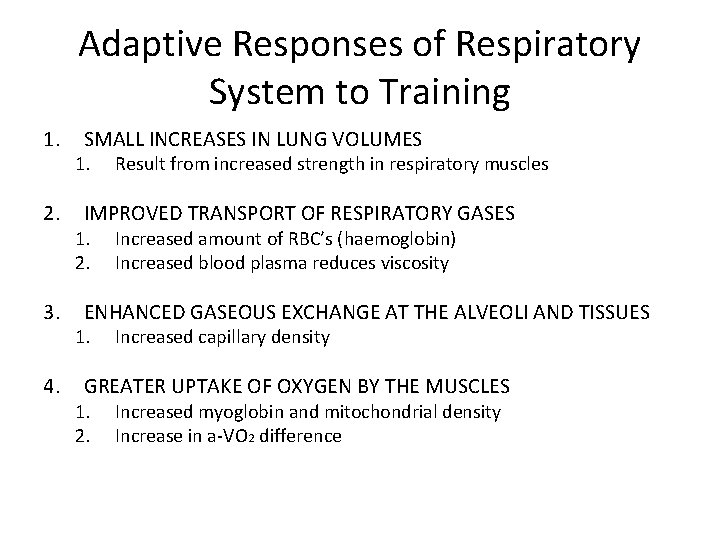
- Slides: 56
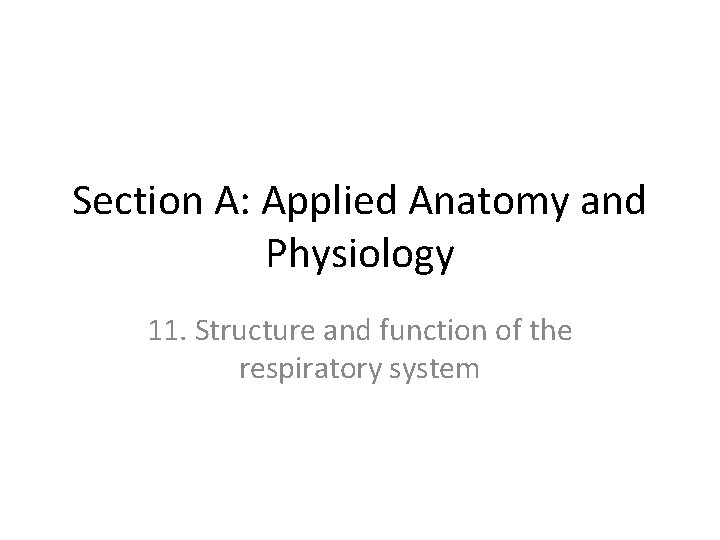
Section A: Applied Anatomy and Physiology 11. Structure and function of the respiratory system
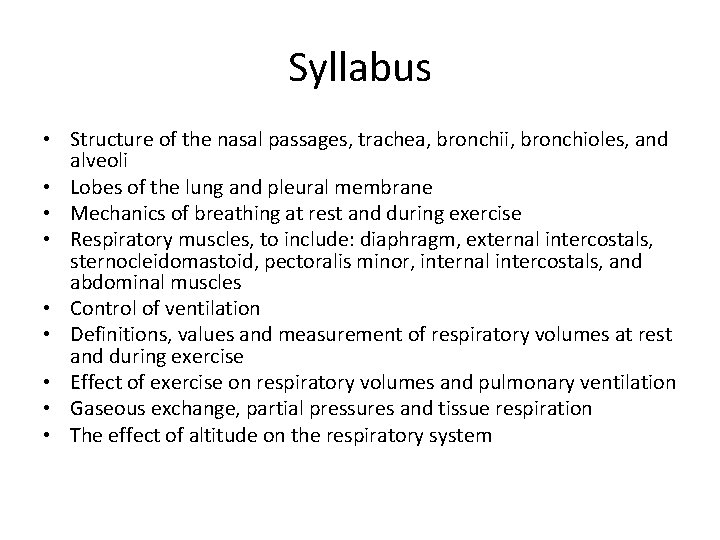
Syllabus • Structure of the nasal passages, trachea, bronchii, bronchioles, and alveoli • Lobes of the lung and pleural membrane • Mechanics of breathing at rest and during exercise • Respiratory muscles, to include: diaphragm, external intercostals, sternocleidomastoid, pectoralis minor, internal intercostals, and abdominal muscles • Control of ventilation • Definitions, values and measurement of respiratory volumes at rest and during exercise • Effect of exercise on respiratory volumes and pulmonary ventilation • Gaseous exchange, partial pressures and tissue respiration • The effect of altitude on the respiratory system
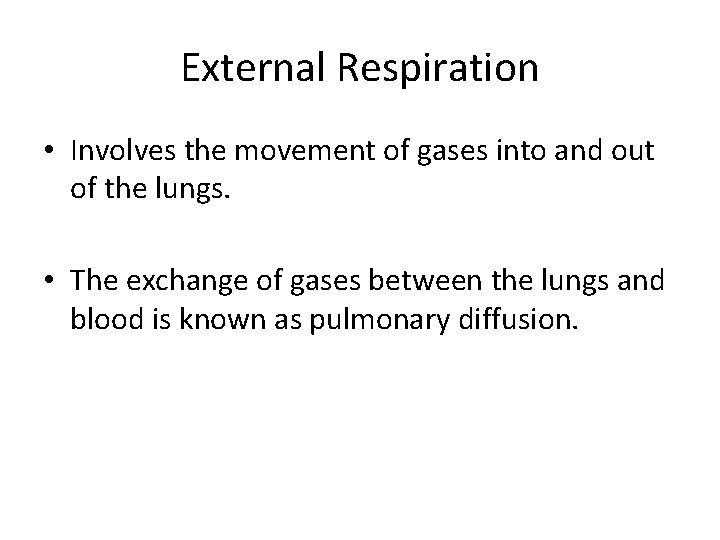
External Respiration • Involves the movement of gases into and out of the lungs. • The exchange of gases between the lungs and blood is known as pulmonary diffusion.
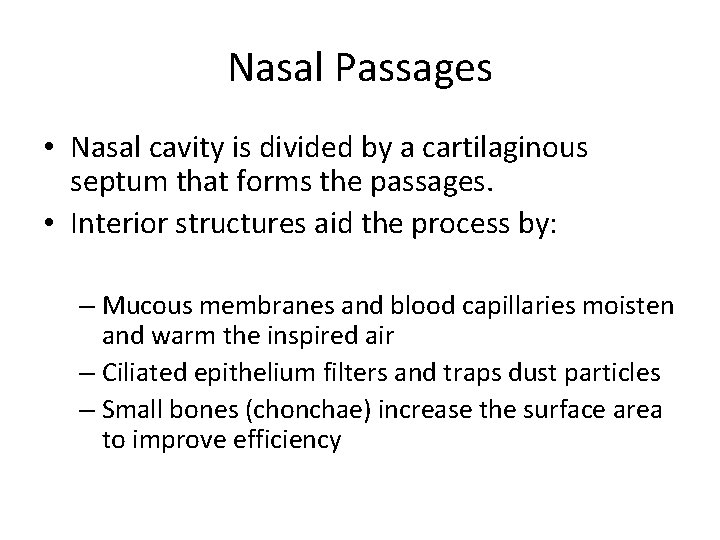
Nasal Passages • Nasal cavity is divided by a cartilaginous septum that forms the passages. • Interior structures aid the process by: – Mucous membranes and blood capillaries moisten and warm the inspired air – Ciliated epithelium filters and traps dust particles – Small bones (chonchae) increase the surface area to improve efficiency
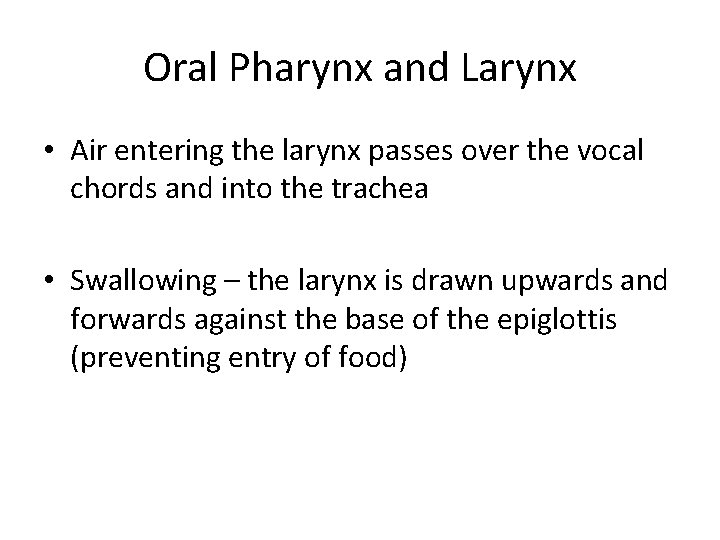
Oral Pharynx and Larynx • Air entering the larynx passes over the vocal chords and into the trachea • Swallowing – the larynx is drawn upwards and forwards against the base of the epiglottis (preventing entry of food)
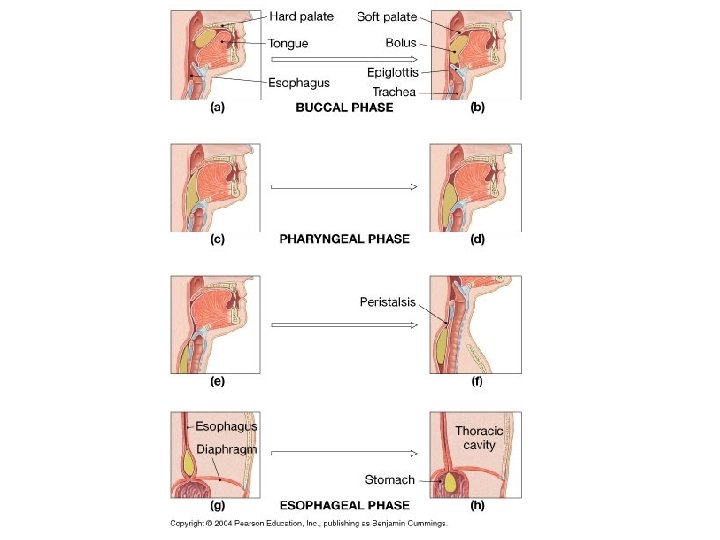
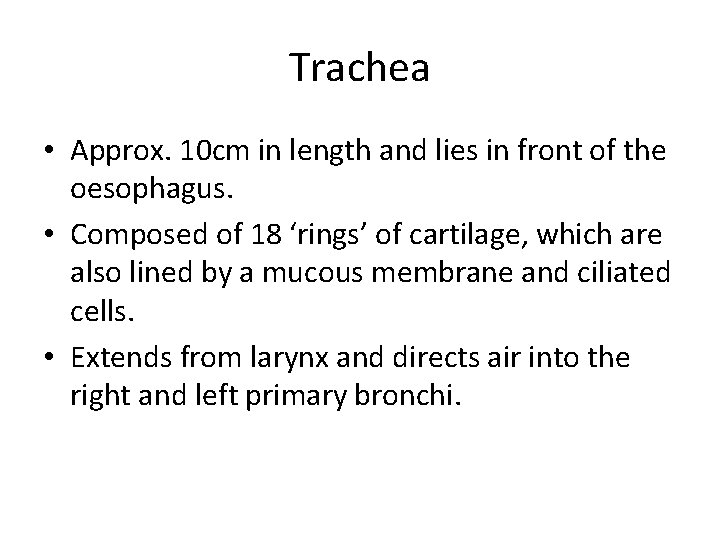
Trachea • Approx. 10 cm in length and lies in front of the oesophagus. • Composed of 18 ‘rings’ of cartilage, which are also lined by a mucous membrane and ciliated cells. • Extends from larynx and directs air into the right and left primary bronchi.
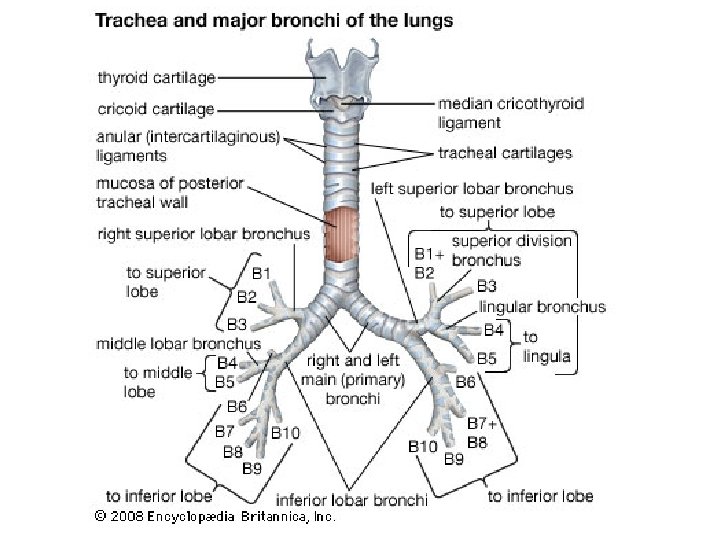
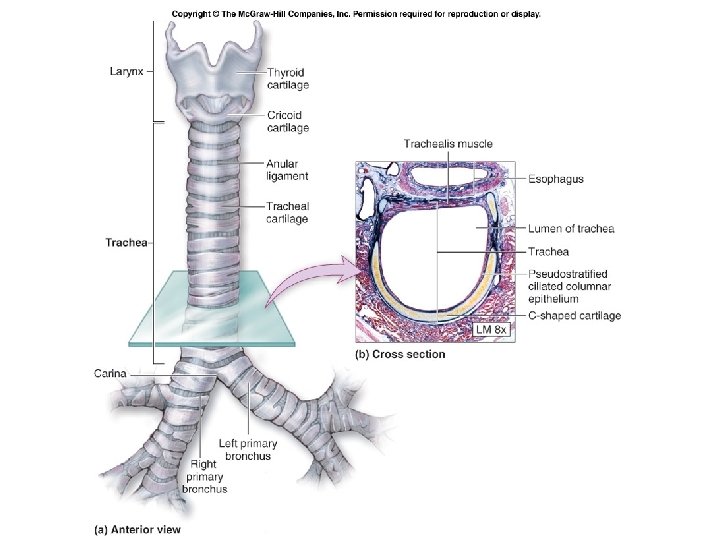
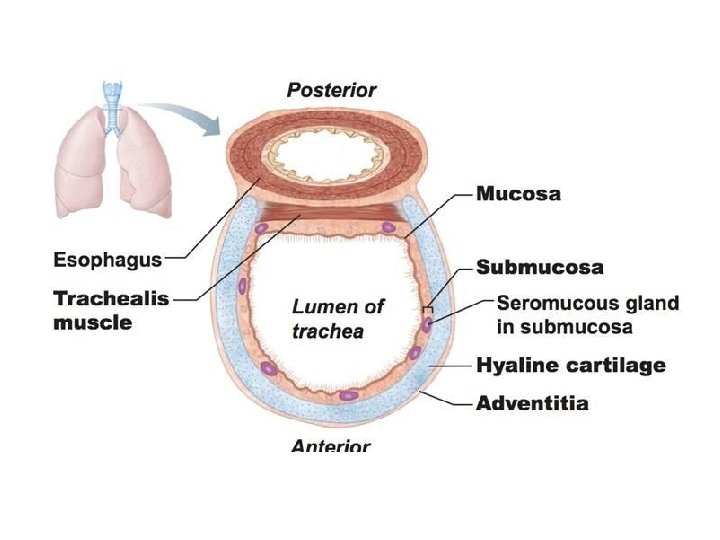
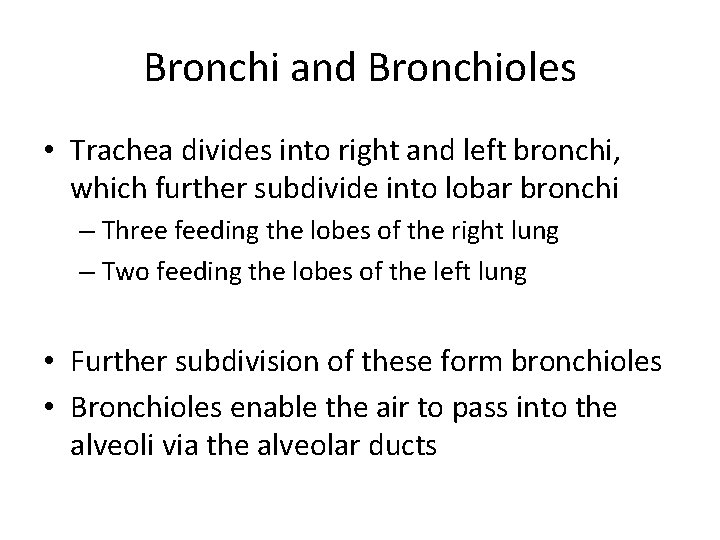
Bronchi and Bronchioles • Trachea divides into right and left bronchi, which further subdivide into lobar bronchi – Three feeding the lobes of the right lung – Two feeding the lobes of the left lung • Further subdivision of these form bronchioles • Bronchioles enable the air to pass into the alveoli via the alveolar ducts
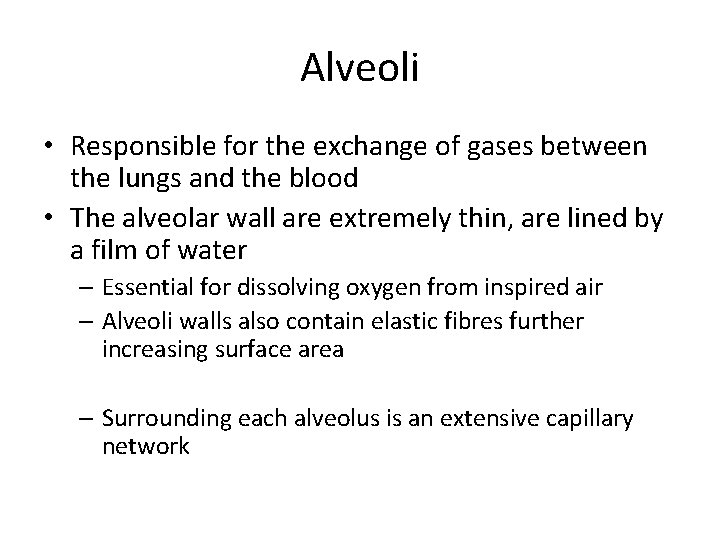
Alveoli • Responsible for the exchange of gases between the lungs and the blood • The alveolar wall are extremely thin, are lined by a film of water – Essential for dissolving oxygen from inspired air – Alveoli walls also contain elastic fibres further increasing surface area – Surrounding each alveolus is an extensive capillary network
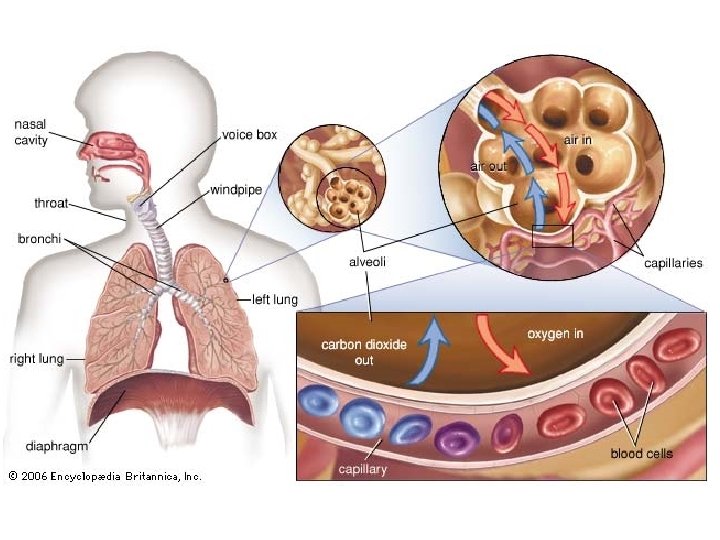
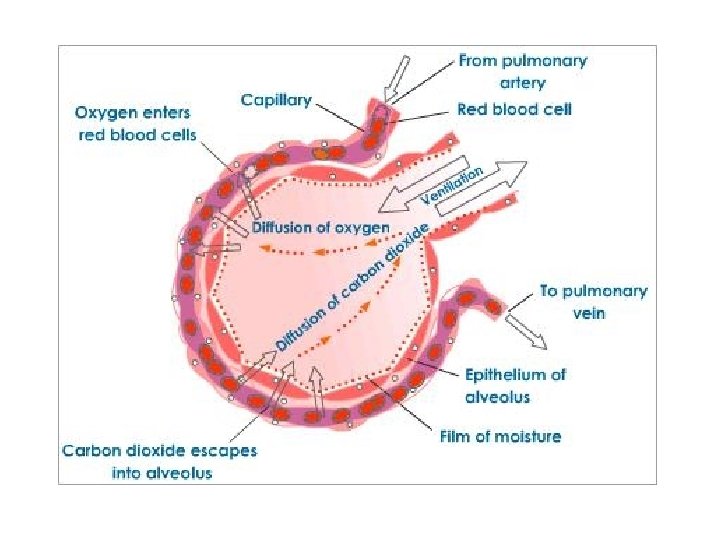
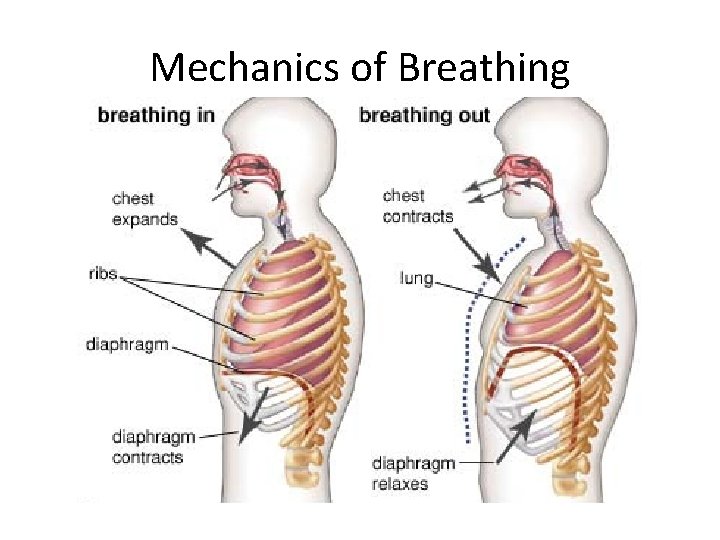
Mechanics of Breathing
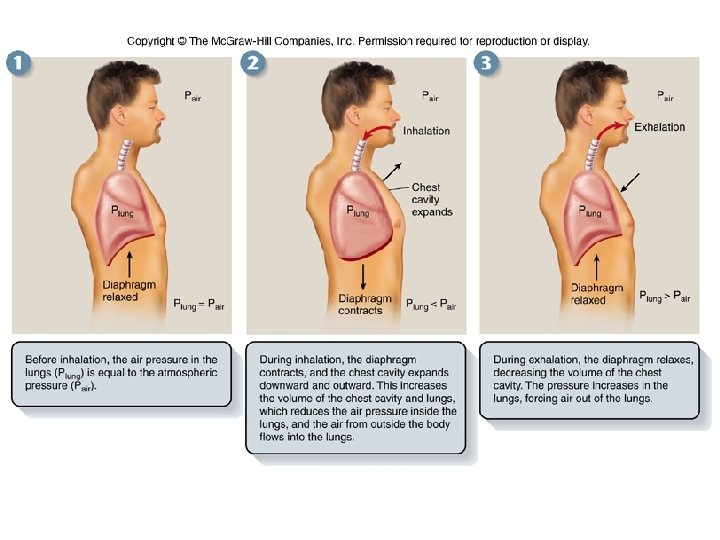
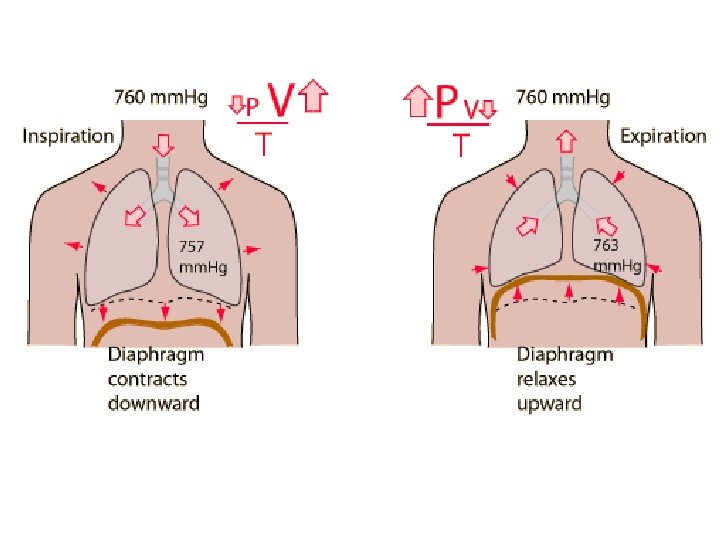
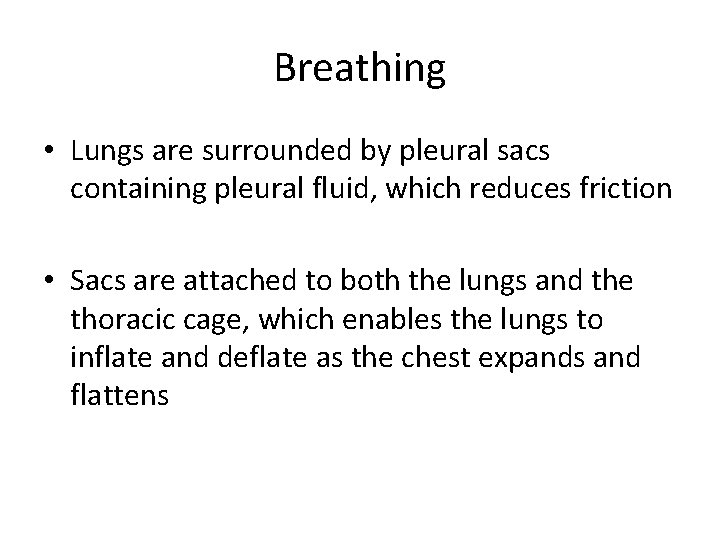
Breathing • Lungs are surrounded by pleural sacs containing pleural fluid, which reduces friction • Sacs are attached to both the lungs and the thoracic cage, which enables the lungs to inflate and deflate as the chest expands and flattens
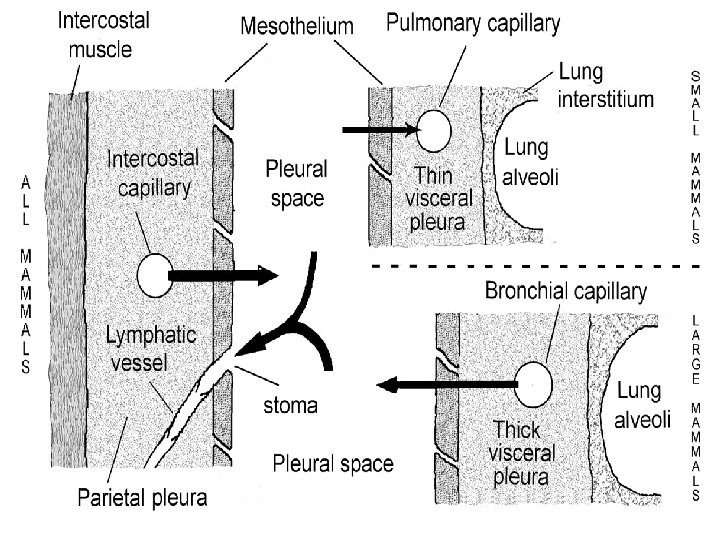
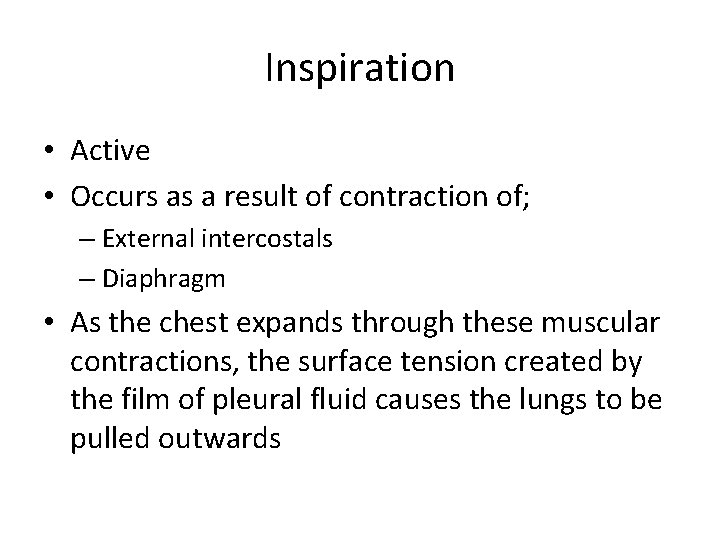
Inspiration • Active • Occurs as a result of contraction of; – External intercostals – Diaphragm • As the chest expands through these muscular contractions, the surface tension created by the film of pleural fluid causes the lungs to be pulled outwards
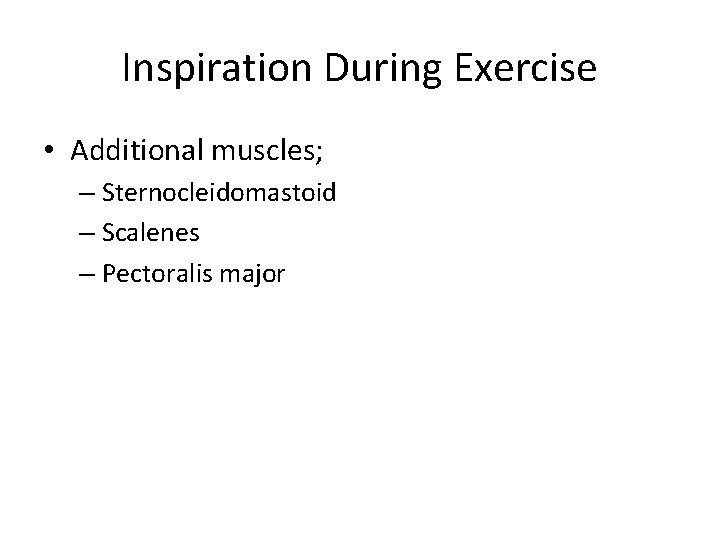
Inspiration During Exercise • Additional muscles; – Sternocleidomastoid – Scalenes – Pectoralis major
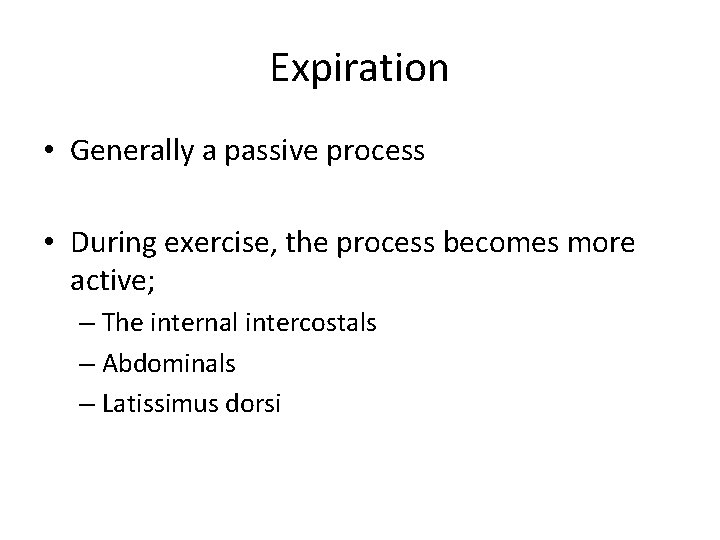
Expiration • Generally a passive process • During exercise, the process becomes more active; – The internal intercostals – Abdominals – Latissimus dorsi
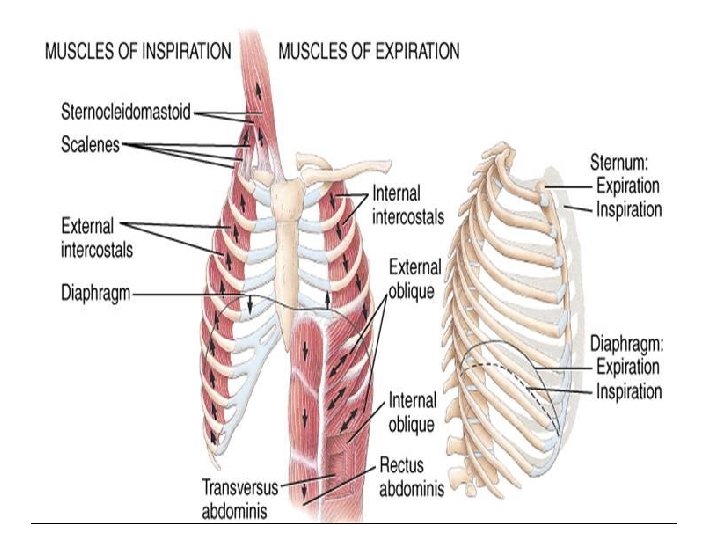
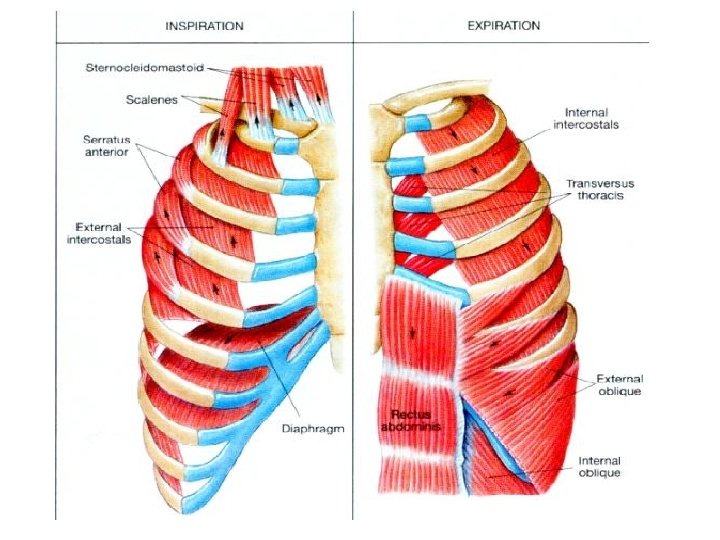
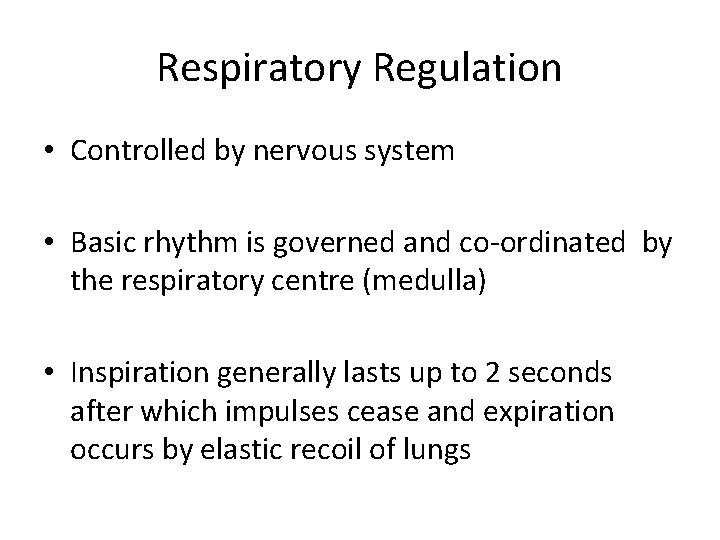
Respiratory Regulation • Controlled by nervous system • Basic rhythm is governed and co-ordinated by the respiratory centre (medulla) • Inspiration generally lasts up to 2 seconds after which impulses cease and expiration occurs by elastic recoil of lungs
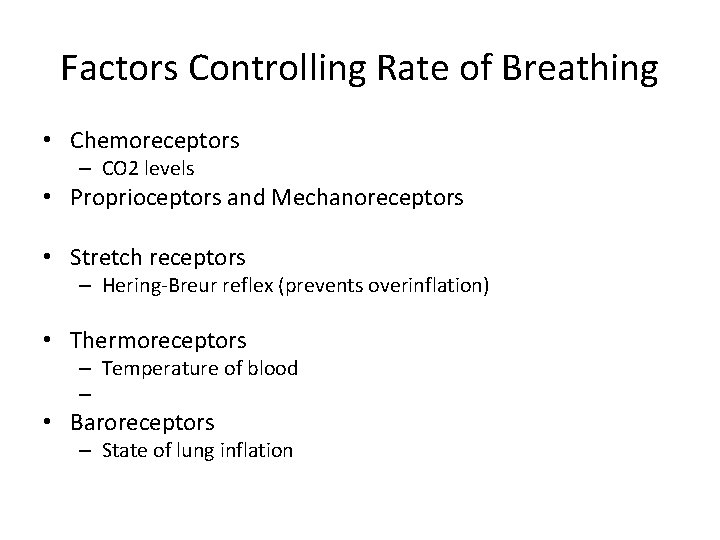
Factors Controlling Rate of Breathing • Chemoreceptors – CO 2 levels • Proprioceptors and Mechanoreceptors • Stretch receptors – Hering-Breur reflex (prevents overinflation) • Thermoreceptors – Temperature of blood – • Baroreceptors – State of lung inflation
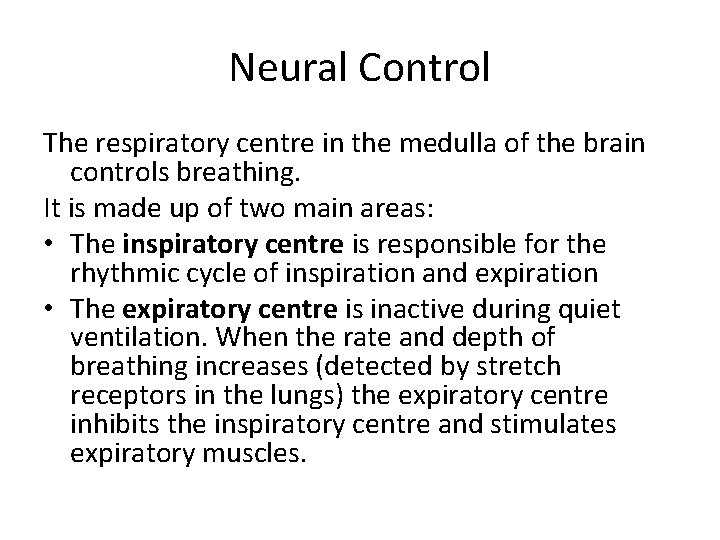
Neural Control The respiratory centre in the medulla of the brain controls breathing. It is made up of two main areas: • The inspiratory centre is responsible for the rhythmic cycle of inspiration and expiration • The expiratory centre is inactive during quiet ventilation. When the rate and depth of breathing increases (detected by stretch receptors in the lungs) the expiratory centre inhibits the inspiratory centre and stimulates expiratory muscles.
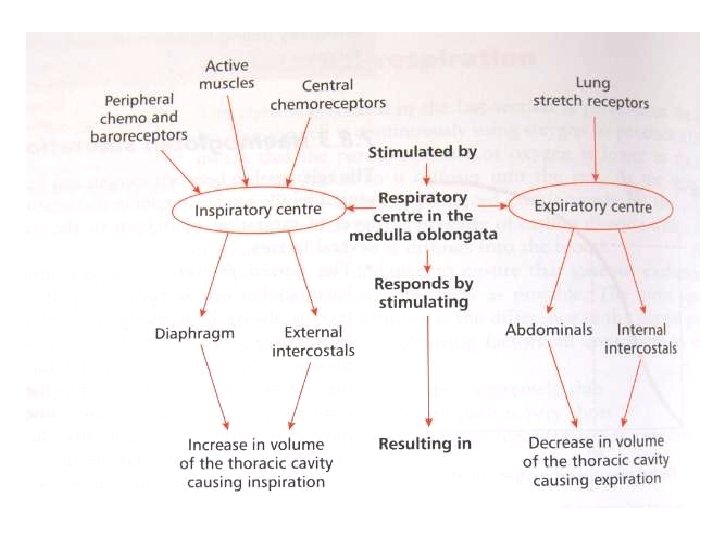
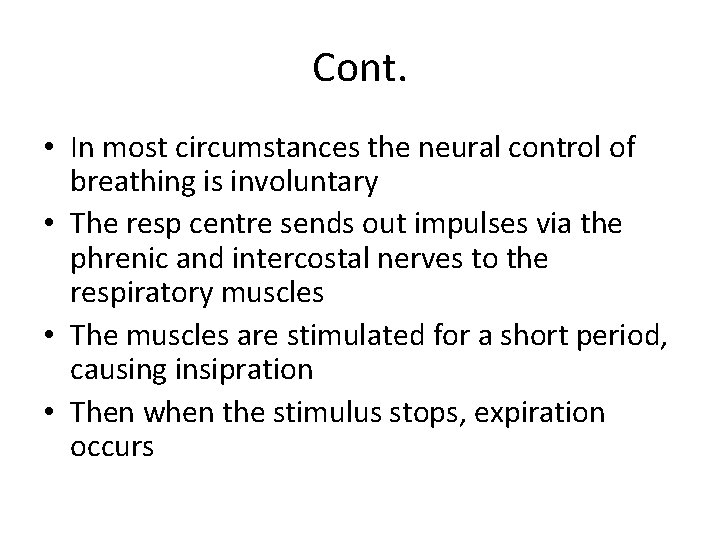
Cont. • In most circumstances the neural control of breathing is involuntary • The resp centre sends out impulses via the phrenic and intercostal nerves to the respiratory muscles • The muscles are stimulated for a short period, causing insipration • Then when the stimulus stops, expiration occurs
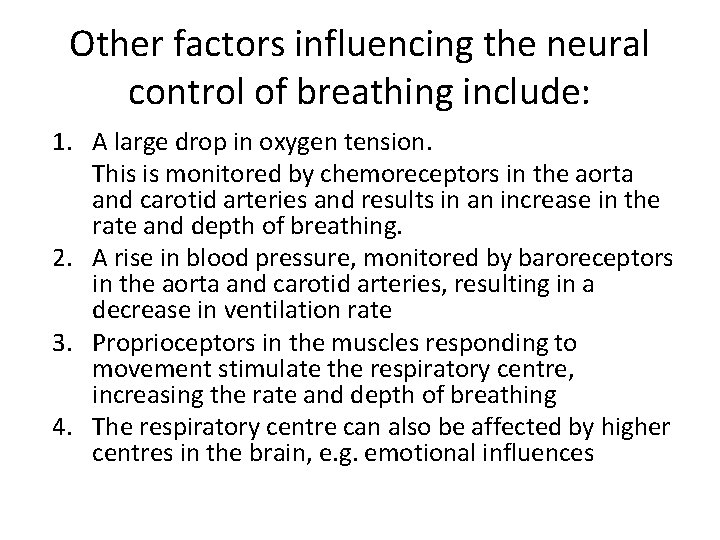
Other factors influencing the neural control of breathing include: 1. A large drop in oxygen tension. This is monitored by chemoreceptors in the aorta and carotid arteries and results in an increase in the rate and depth of breathing. 2. A rise in blood pressure, monitored by baroreceptors in the aorta and carotid arteries, resulting in a decrease in ventilation rate 3. Proprioceptors in the muscles responding to movement stimulate the respiratory centre, increasing the rate and depth of breathing 4. The respiratory centre can also be affected by higher centres in the brain, e. g. emotional influences
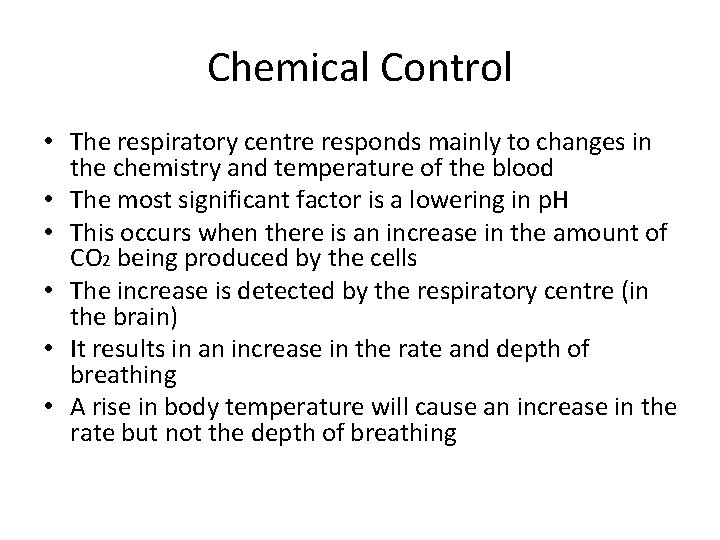
Chemical Control • The respiratory centre responds mainly to changes in the chemistry and temperature of the blood • The most significant factor is a lowering in p. H • This occurs when there is an increase in the amount of CO 2 being produced by the cells • The increase is detected by the respiratory centre (in the brain) • It results in an increase in the rate and depth of breathing • A rise in body temperature will cause an increase in the rate but not the depth of breathing
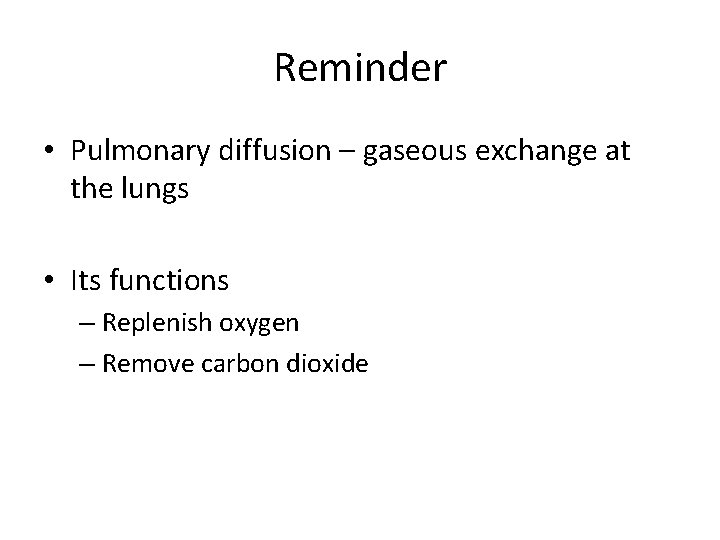
Reminder • Pulmonary diffusion – gaseous exchange at the lungs • Its functions – Replenish oxygen – Remove carbon dioxide
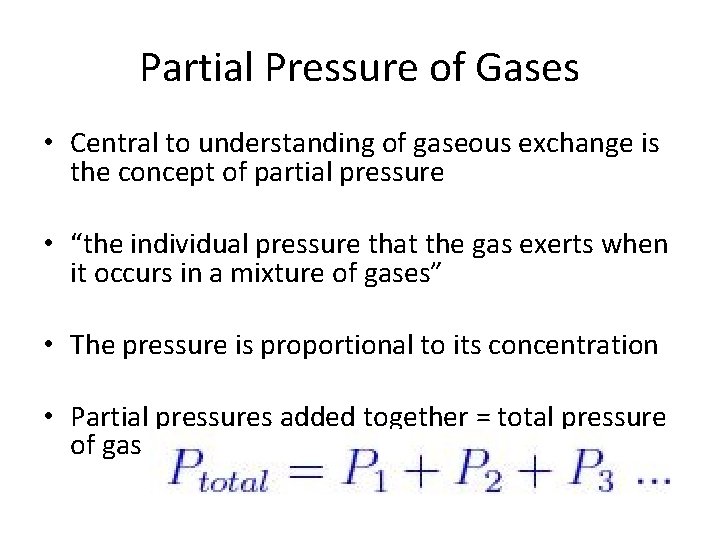
Partial Pressure of Gases • Central to understanding of gaseous exchange is the concept of partial pressure • “the individual pressure that the gas exerts when it occurs in a mixture of gases” • The pressure is proportional to its concentration • Partial pressures added together = total pressure of gas
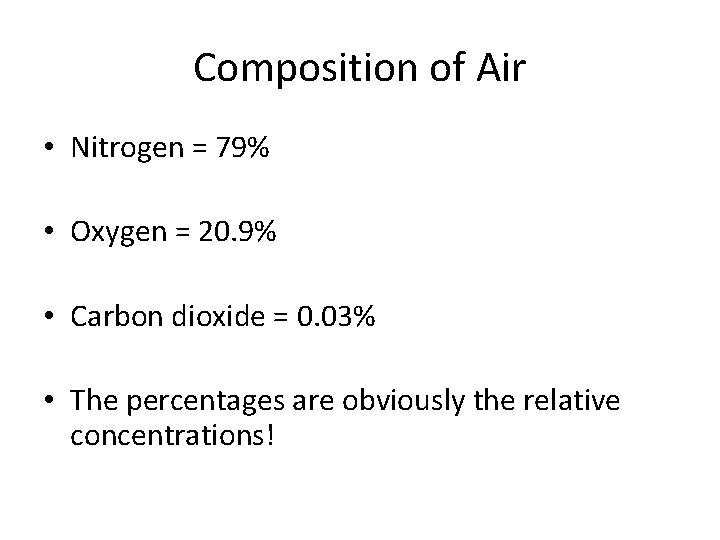
Composition of Air • Nitrogen = 79% • Oxygen = 20. 9% • Carbon dioxide = 0. 03% • The percentages are obviously the relative concentrations!
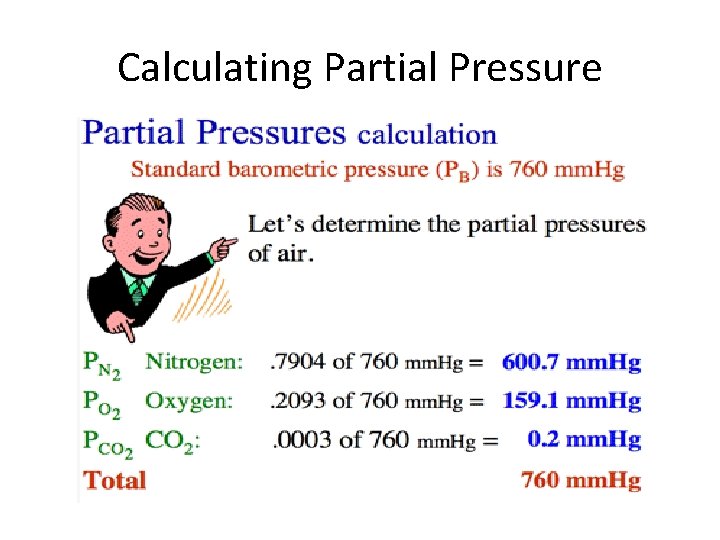
Calculating Partial Pressure
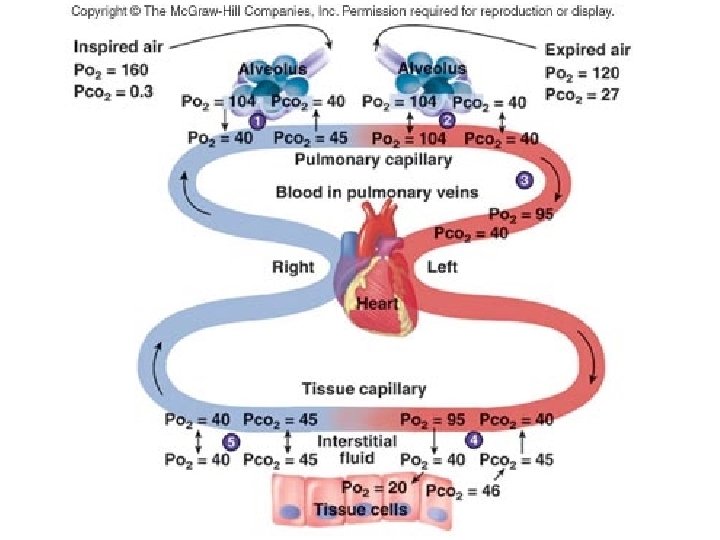
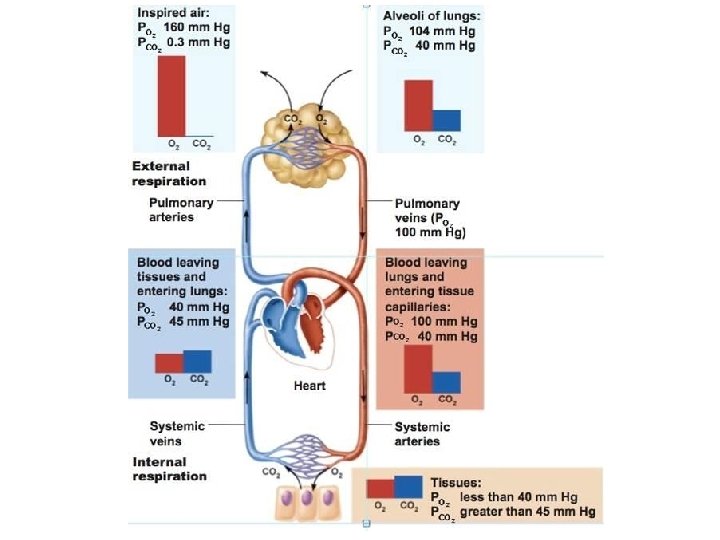
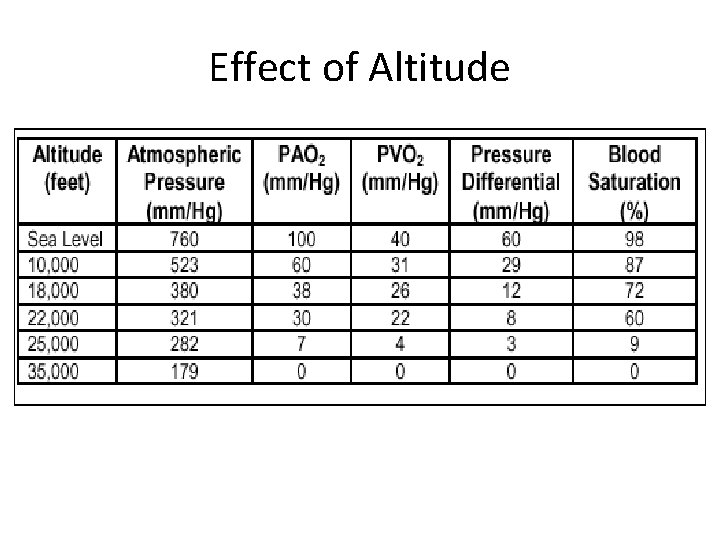
Effect of Altitude
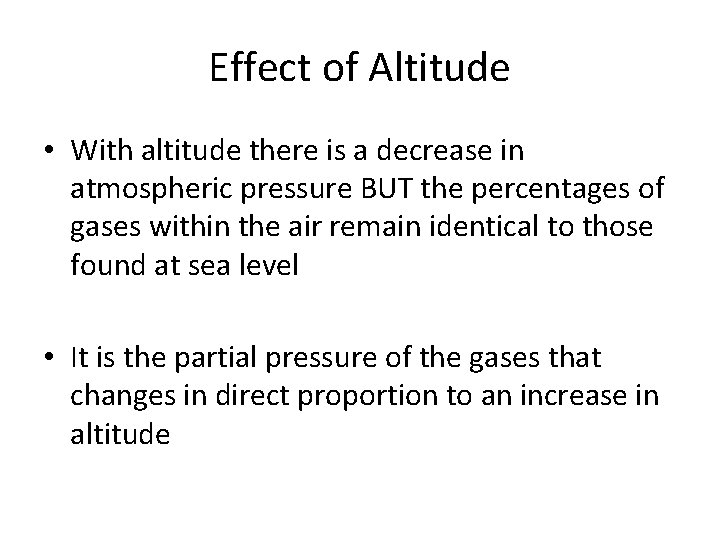
Effect of Altitude • With altitude there is a decrease in atmospheric pressure BUT the percentages of gases within the air remain identical to those found at sea level • It is the partial pressure of the gases that changes in direct proportion to an increase in altitude
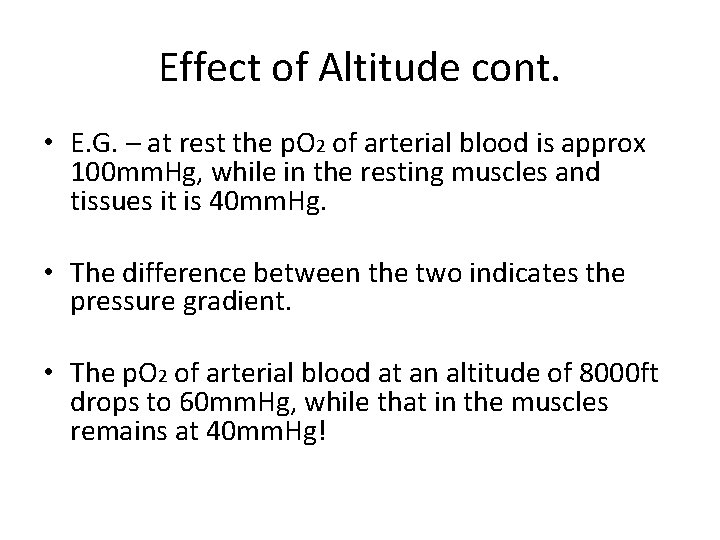
Effect of Altitude cont. • E. G. – at rest the p. O 2 of arterial blood is approx 100 mm. Hg, while in the resting muscles and tissues it is 40 mm. Hg. • The difference between the two indicates the pressure gradient. • The p. O 2 of arterial blood at an altitude of 8000 ft drops to 60 mm. Hg, while that in the muscles remains at 40 mm. Hg!
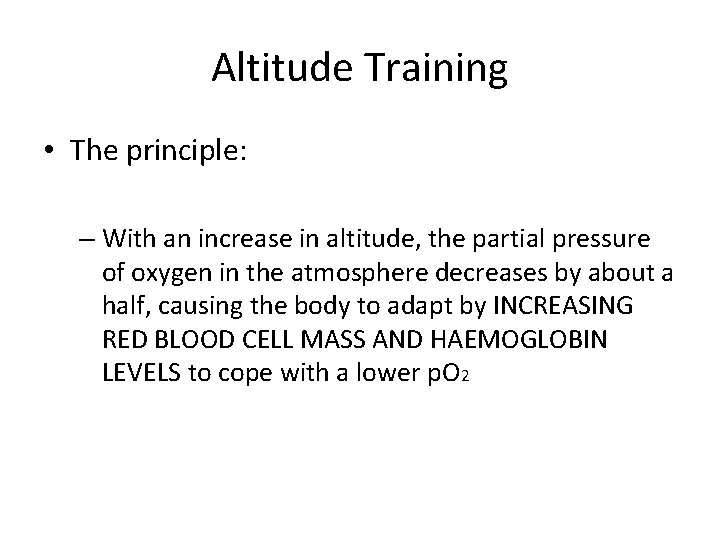
Altitude Training • The principle: – With an increase in altitude, the partial pressure of oxygen in the atmosphere decreases by about a half, causing the body to adapt by INCREASING RED BLOOD CELL MASS AND HAEMOGLOBIN LEVELS to cope with a lower p. O 2
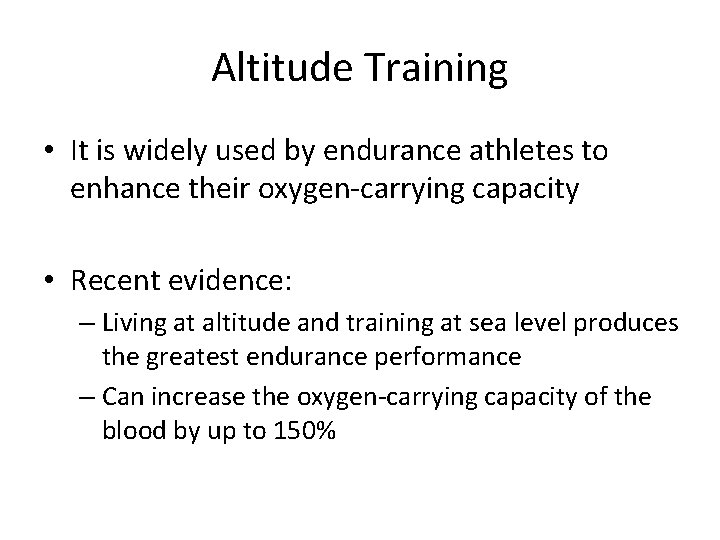
Altitude Training • It is widely used by endurance athletes to enhance their oxygen-carrying capacity • Recent evidence: – Living at altitude and training at sea level produces the greatest endurance performance – Can increase the oxygen-carrying capacity of the blood by up to 150%
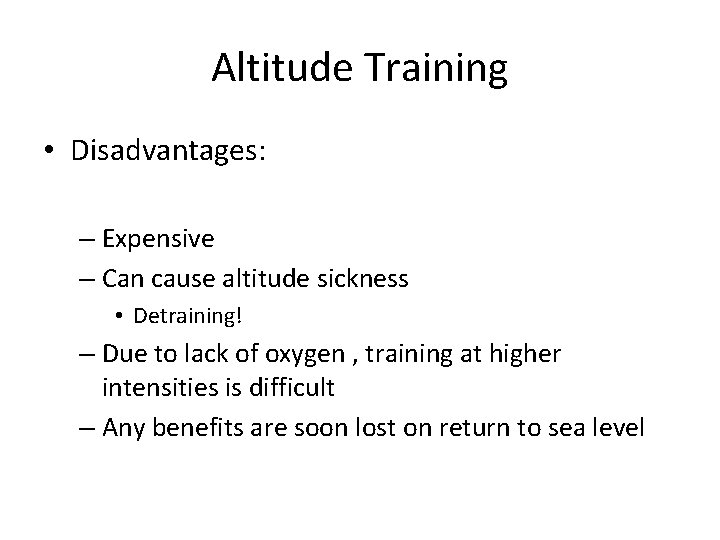
Altitude Training • Disadvantages: – Expensive – Can cause altitude sickness • Detraining! – Due to lack of oxygen , training at higher intensities is difficult – Any benefits are soon lost on return to sea level
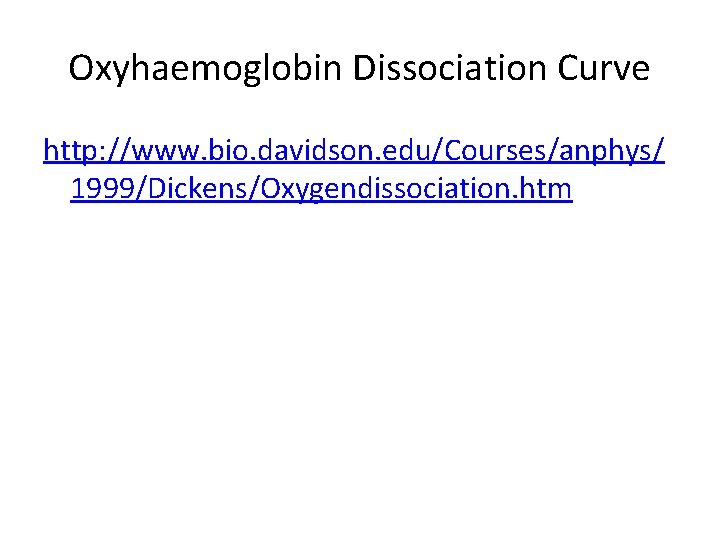
Oxyhaemoglobin Dissociation Curve http: //www. bio. davidson. edu/Courses/anphys/ 1999/Dickens/Oxygendissociation. htm
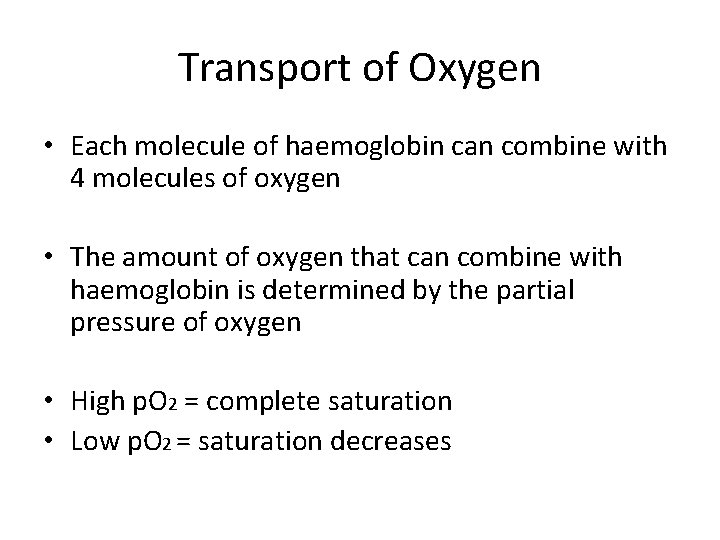
Transport of Oxygen • Each molecule of haemoglobin can combine with 4 molecules of oxygen • The amount of oxygen that can combine with haemoglobin is determined by the partial pressure of oxygen • High p. O 2 = complete saturation • Low p. O 2 = saturation decreases
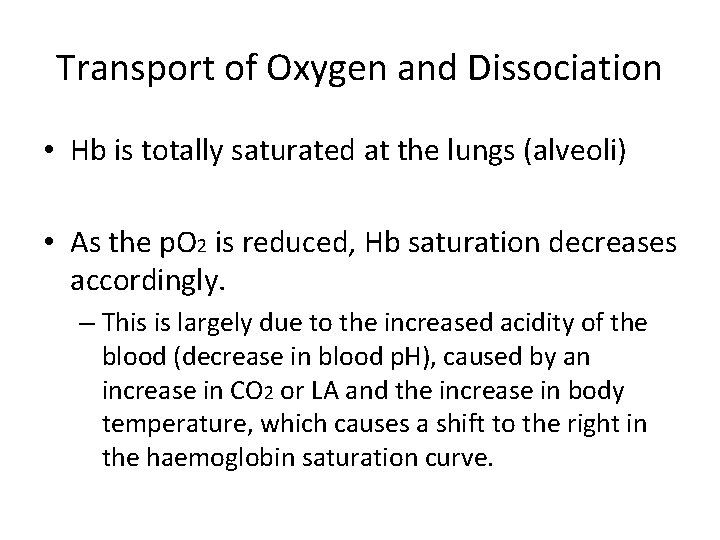
Transport of Oxygen and Dissociation • Hb is totally saturated at the lungs (alveoli) • As the p. O 2 is reduced, Hb saturation decreases accordingly. – This is largely due to the increased acidity of the blood (decrease in blood p. H), caused by an increase in CO 2 or LA and the increase in body temperature, which causes a shift to the right in the haemoglobin saturation curve.
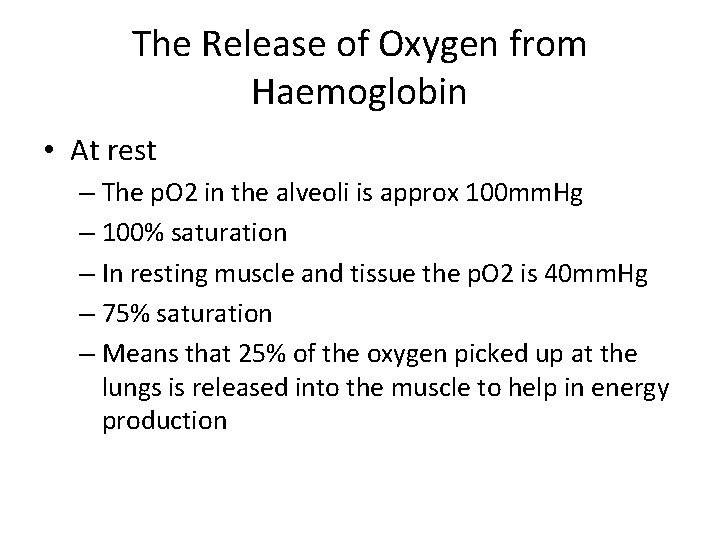
The Release of Oxygen from Haemoglobin • At rest – The p. O 2 in the alveoli is approx 100 mm. Hg – 100% saturation – In resting muscle and tissue the p. O 2 is 40 mm. Hg – 75% saturation – Means that 25% of the oxygen picked up at the lungs is released into the muscle to help in energy production
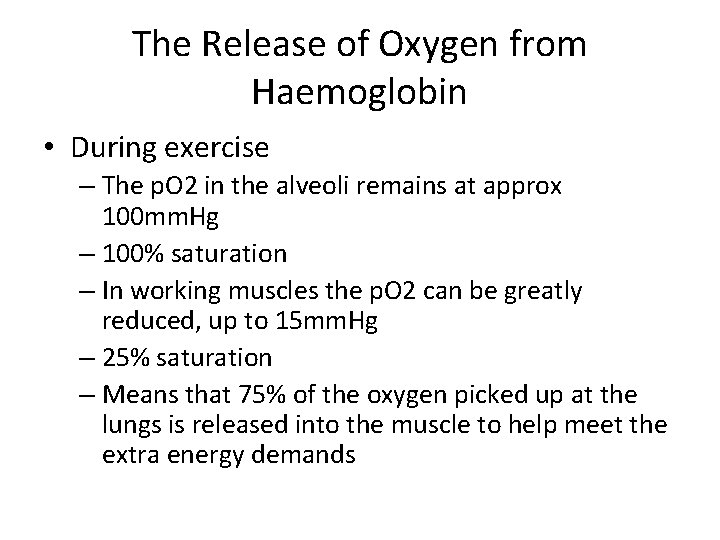
The Release of Oxygen from Haemoglobin • During exercise – The p. O 2 in the alveoli remains at approx 100 mm. Hg – 100% saturation – In working muscles the p. O 2 can be greatly reduced, up to 15 mm. Hg – 25% saturation – Means that 75% of the oxygen picked up at the lungs is released into the muscle to help meet the extra energy demands
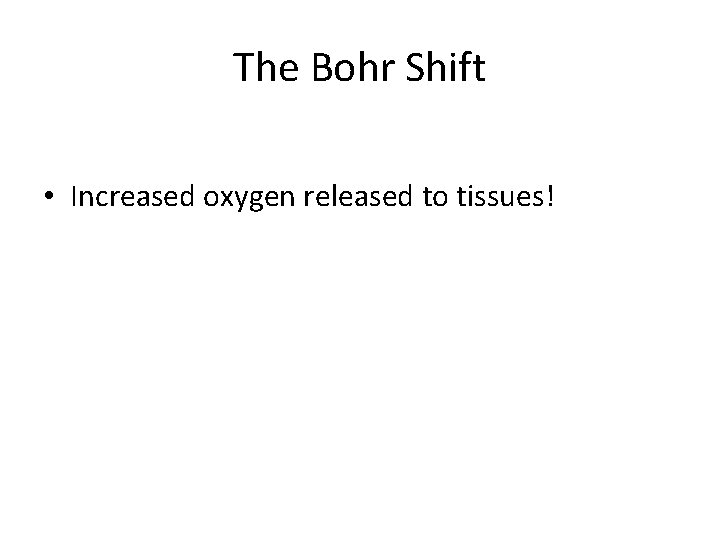
The Bohr Shift • Increased oxygen released to tissues!
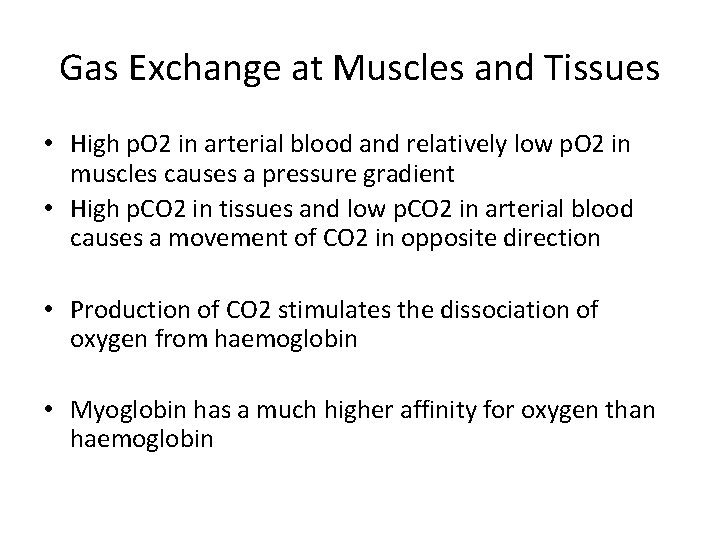
Gas Exchange at Muscles and Tissues • High p. O 2 in arterial blood and relatively low p. O 2 in muscles causes a pressure gradient • High p. CO 2 in tissues and low p. CO 2 in arterial blood causes a movement of CO 2 in opposite direction • Production of CO 2 stimulates the dissociation of oxygen from haemoglobin • Myoglobin has a much higher affinity for oxygen than haemoglobin
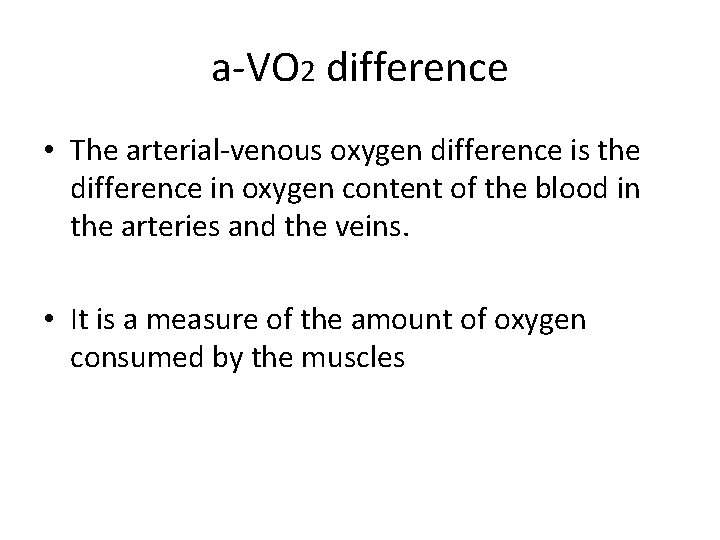
a-VO 2 difference • The arterial-venous oxygen difference is the difference in oxygen content of the blood in the arteries and the veins. • It is a measure of the amount of oxygen consumed by the muscles
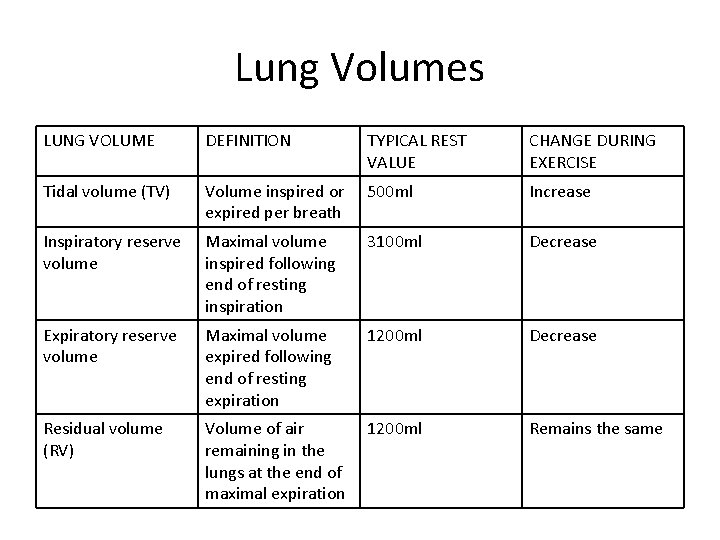
Lung Volumes LUNG VOLUME DEFINITION TYPICAL REST VALUE CHANGE DURING EXERCISE Tidal volume (TV) Volume inspired or expired per breath 500 ml Increase Inspiratory reserve volume Maximal volume inspired following end of resting inspiration 3100 ml Decrease Expiratory reserve volume Maximal volume expired following end of resting expiration 1200 ml Decrease Residual volume (RV) Volume of air remaining in the lungs at the end of maximal expiration 1200 ml Remains the same
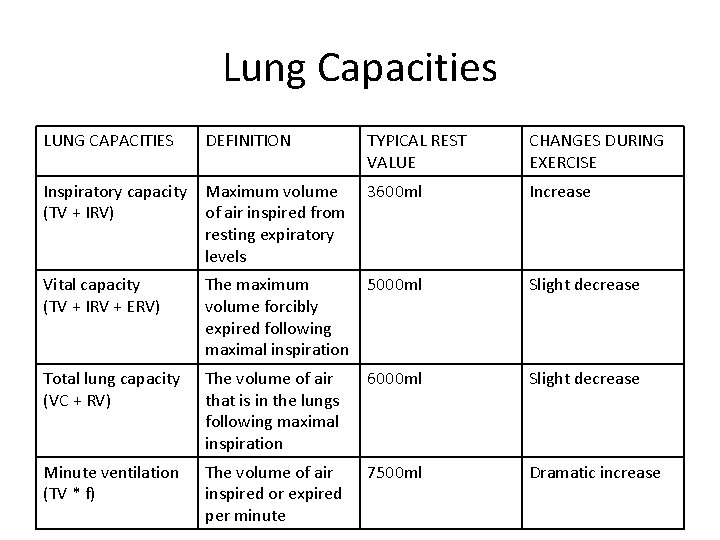
Lung Capacities LUNG CAPACITIES DEFINITION TYPICAL REST VALUE CHANGES DURING EXERCISE Inspiratory capacity (TV + IRV) Maximum volume of air inspired from resting expiratory levels 3600 ml Increase Vital capacity (TV + IRV + ERV) The maximum 5000 ml volume forcibly expired following maximal inspiration Slight decrease Total lung capacity (VC + RV) The volume of air that is in the lungs following maximal inspiration 6000 ml Slight decrease Minute ventilation (TV * f) The volume of air inspired or expired per minute 7500 ml Dramatic increase
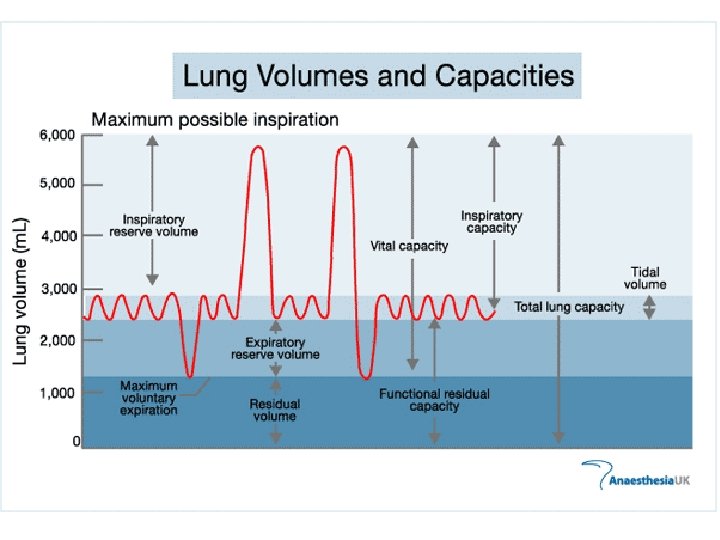
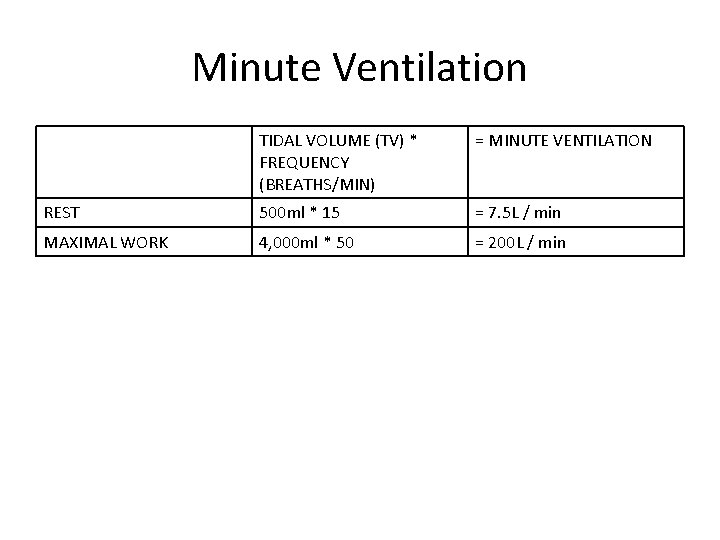
Minute Ventilation TIDAL VOLUME (TV) * FREQUENCY (BREATHS/MIN) = MINUTE VENTILATION REST 500 ml * 15 = 7. 5 L / min MAXIMAL WORK 4, 000 ml * 50 = 200 L / min
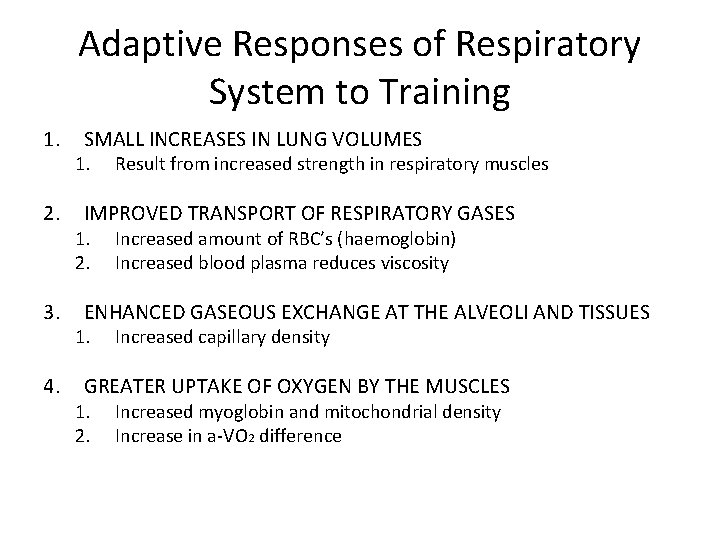
Adaptive Responses of Respiratory System to Training 1. 2. 3. 4. SMALL INCREASES IN LUNG VOLUMES 1. Result from increased strength in respiratory muscles IMPROVED TRANSPORT OF RESPIRATORY GASES 1. 2. Increased amount of RBC’s (haemoglobin) Increased blood plasma reduces viscosity ENHANCED GASEOUS EXCHANGE AT THE ALVEOLI AND TISSUES 1. Increased capillary density GREATER UPTAKE OF OXYGEN BY THE MUSCLES 1. 2. Increased myoglobin and mitochondrial density Increase in a-VO 2 difference
External parts of a leaf
Applied physiology
Parts of the upper respiratory tract
Tattoo anatomy and physiology
Science olympiad anatomy and physiology
Anatomy and physiology bone
Anatomy of an ulcer
Liver hilus
Podbřišek
Wpigastric region
Google image
Chapter 14 anatomy and physiology
Human anatomy and physiology seventh edition marieb
Http://anatomy and physiology
Chapter 1 introduction to human anatomy and physiology
Appendix anatomy and physiology
Aohs foundations of anatomy and physiology 1
Aohs foundations of anatomy and physiology 2
Anatomy and physiology of swine
Anatomy and physiology chapter 8 special senses
Chapter 13 anatomy and physiology of pregnancy
Unit 26 agriscience
Science olympiad forensics cheat sheet
Anatomy and physiology chapter 2
Degluttination
Anatomy and physiology of pancreas
Chapter 7 anatomy and physiology
Art labeling activity: figure 14.1 (3 of 3)
Chapter 10 blood anatomy and physiology
Aohs foundations of anatomy and physiology 1
Aohs foundations of anatomy and physiology 1
What produces bile
Anatomy and physiology chapter 15
Cornell notes for anatomy and physiology
Anatomy and physiology edition 9
Necessary life functions anatomy and physiology
Holes anatomy and physiology chapter 1
Holes essential of human anatomy and physiology
Anatomy and physiology unit 7 cardiovascular system
Anatomy and physiology chapter 15
Anatomy and physiology
Medial lateral distal proximal
The speed at which the body consumes energy
Aohs foundations of anatomy and physiology 1
Cephalic cranial
Anatomy and physiology exam 1
Welcome to anatomy and physiology
Physiology of the foot and ankle
Skin cancer
Pancreas anatomy histology
Anatomy and physiology vocabulary
Anatomy and physiology
Anatomy and physiology
Anatomy and physiology
Anatomy and physiology
Anatomy and physiology
Anatomy and physiology