Physics of Heavy Ion Collisions David Hofman UIC
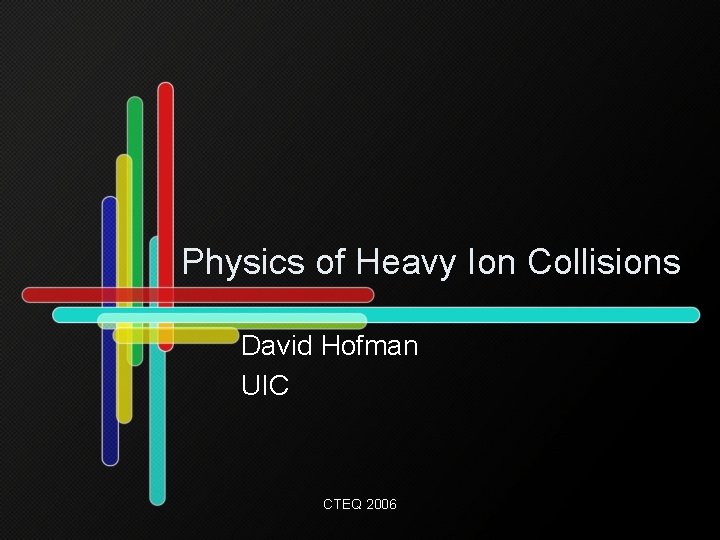
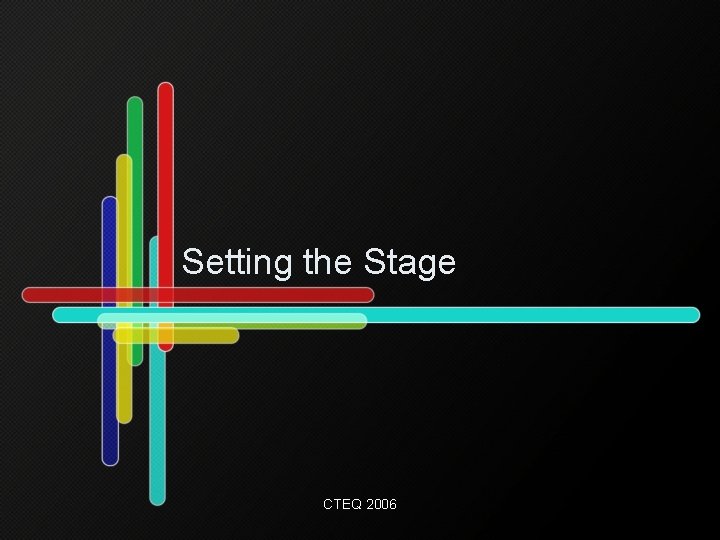
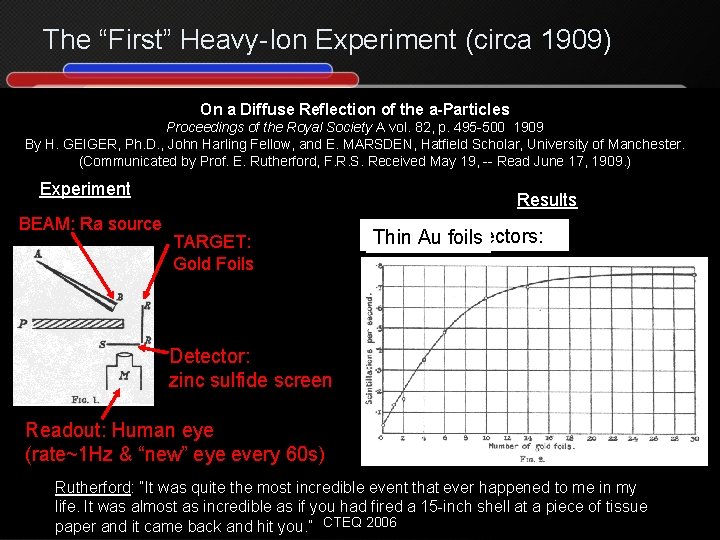
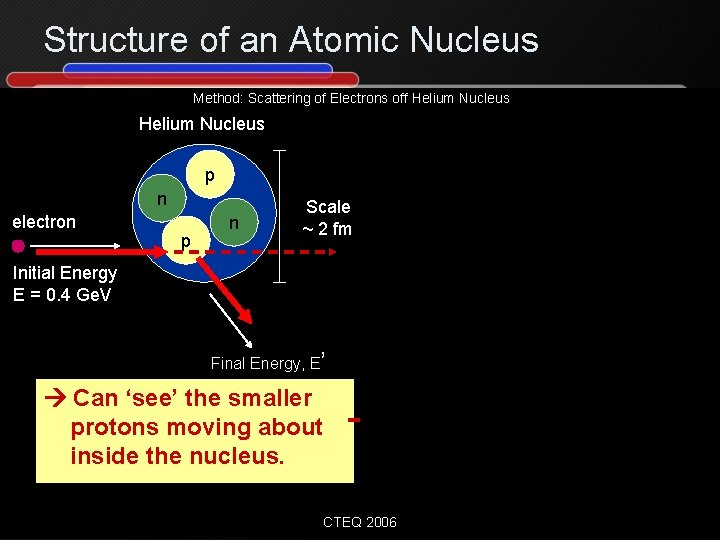
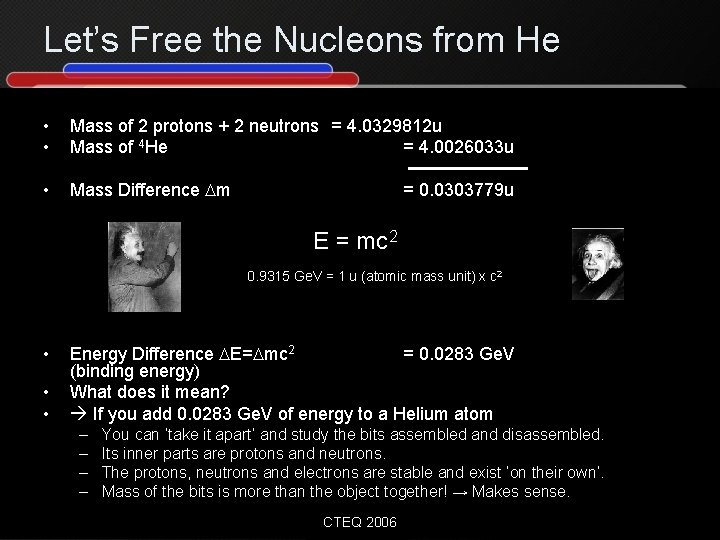
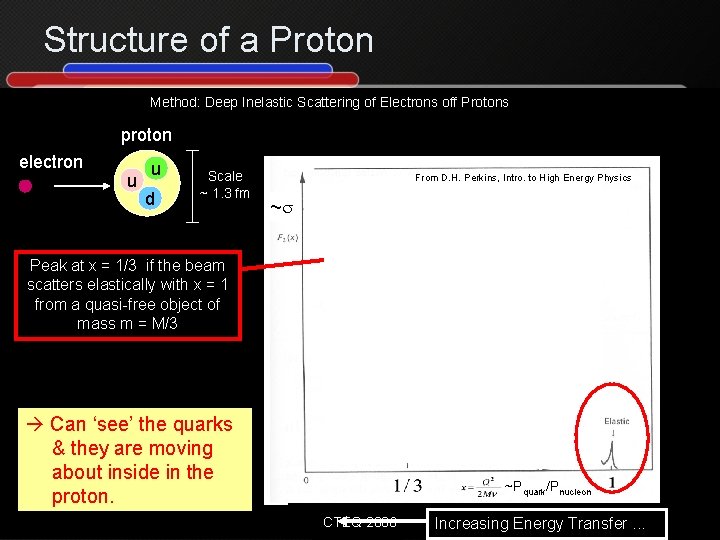
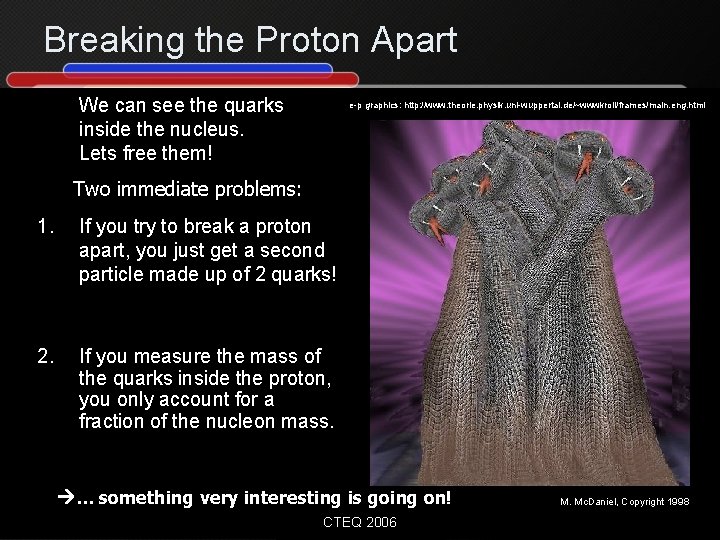
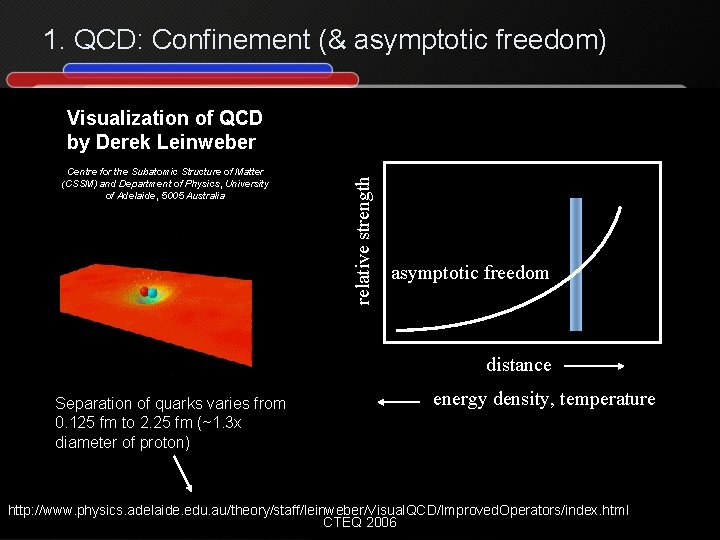
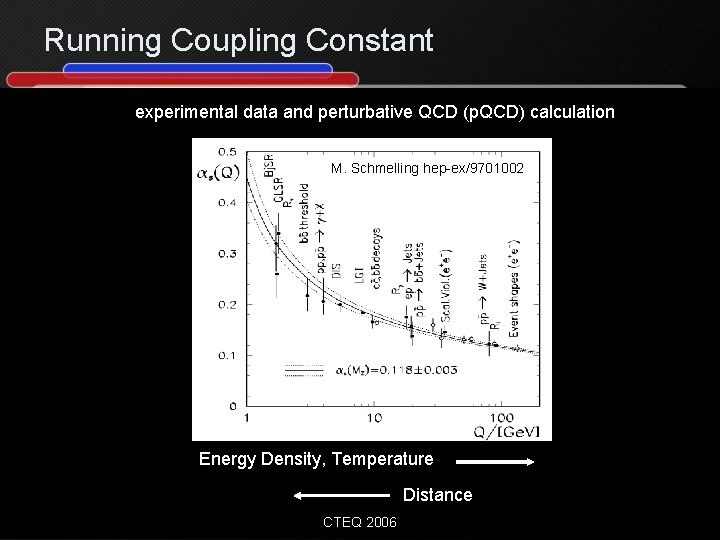
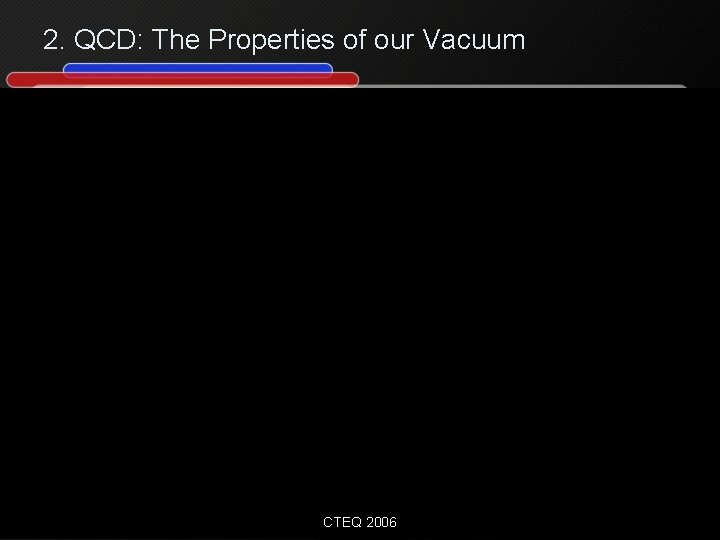
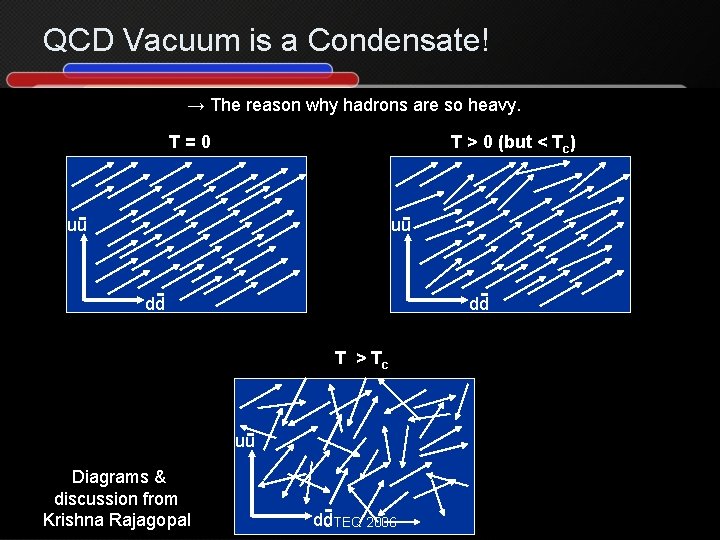
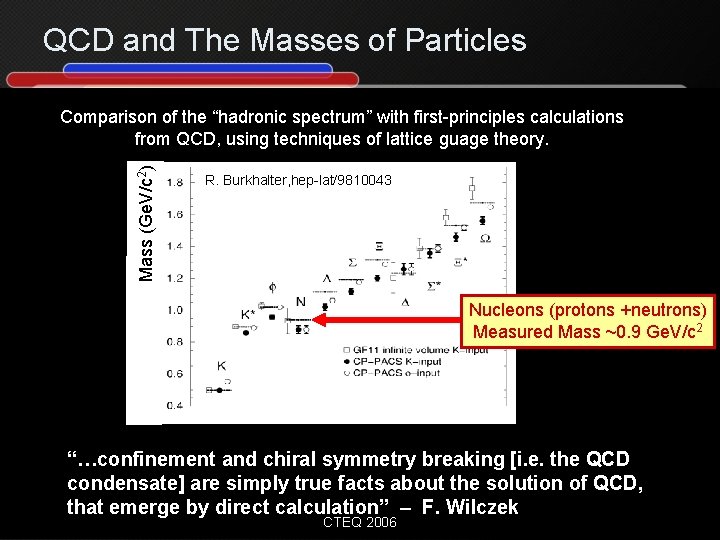
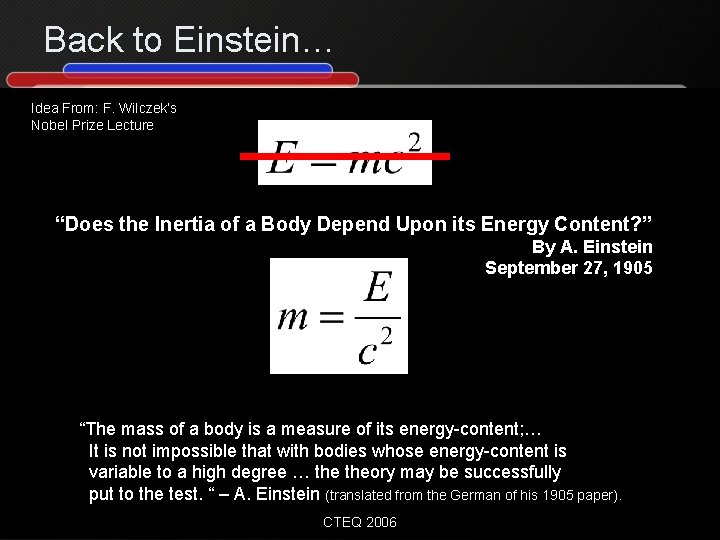
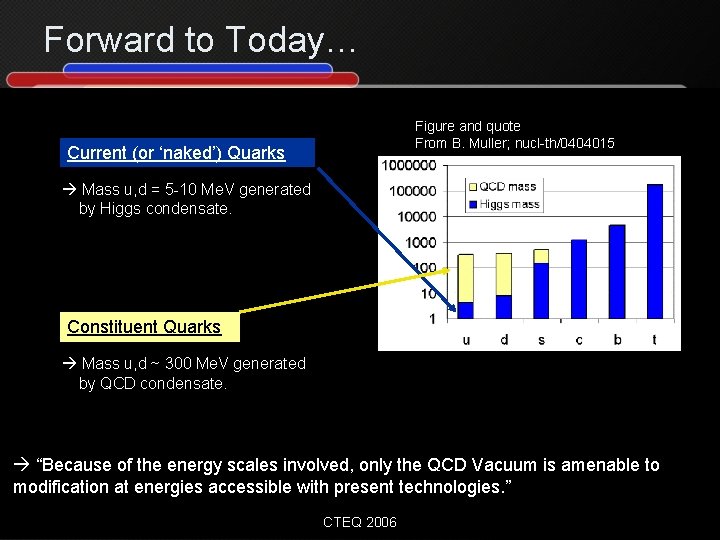
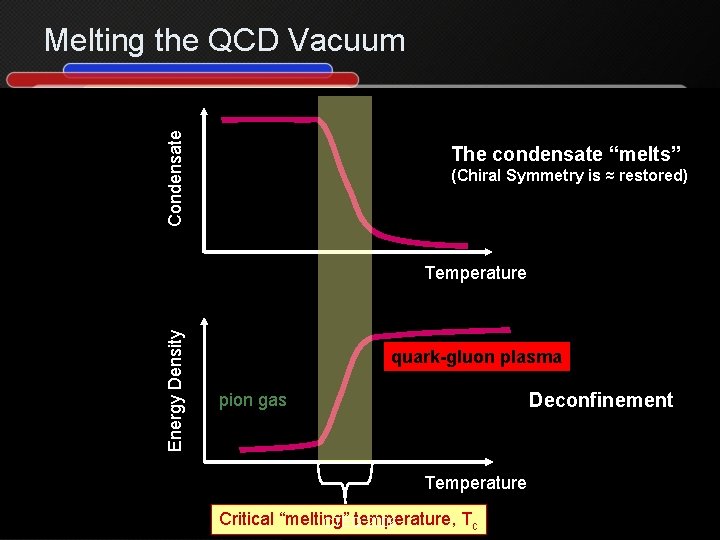
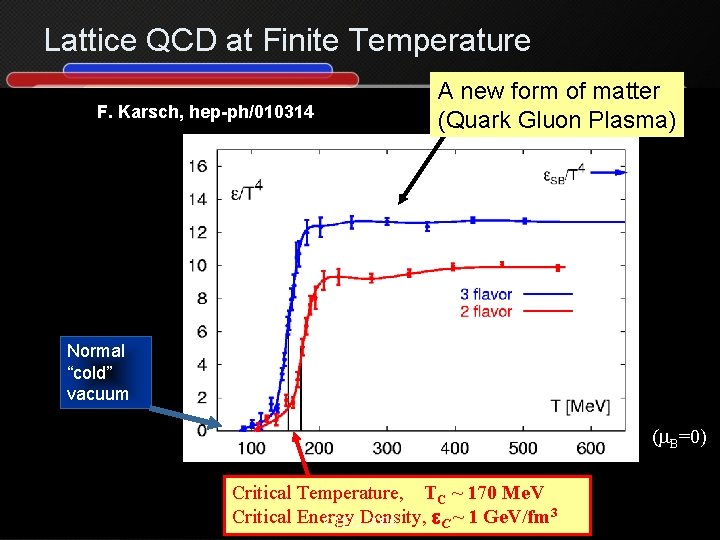
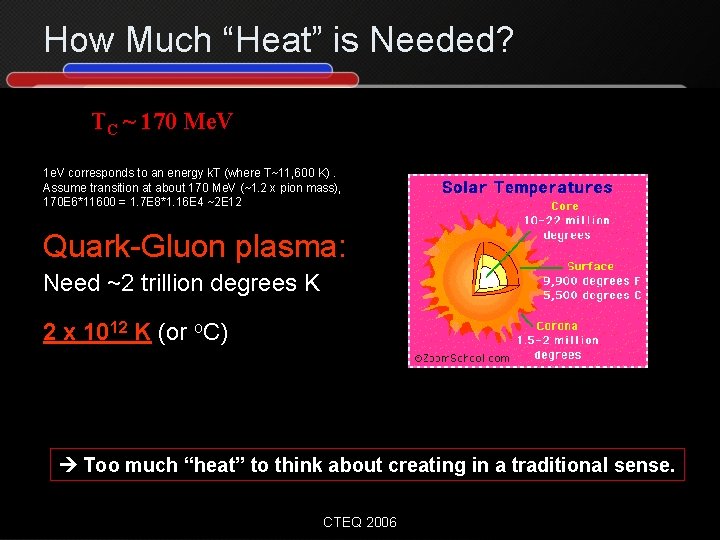
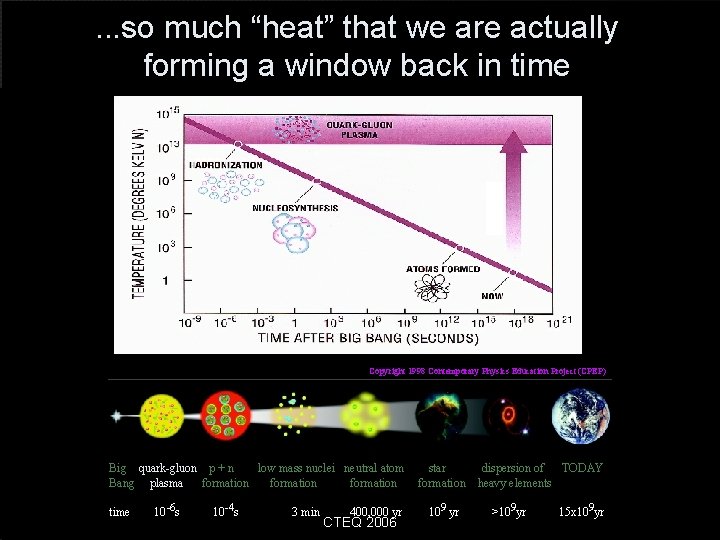
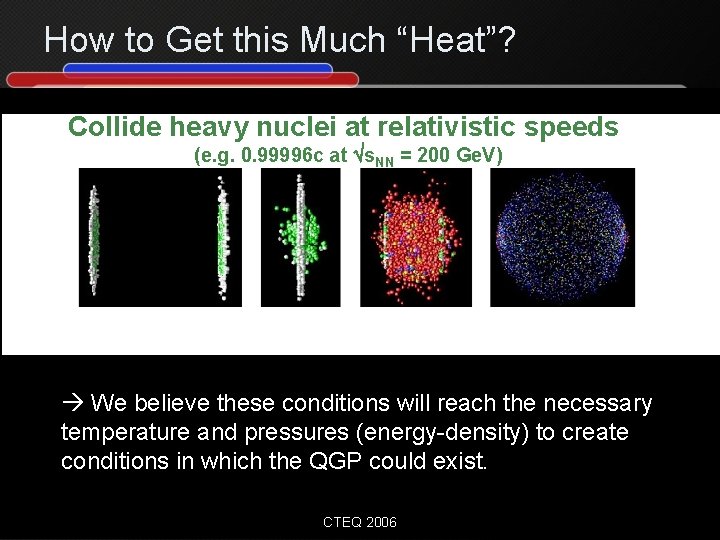
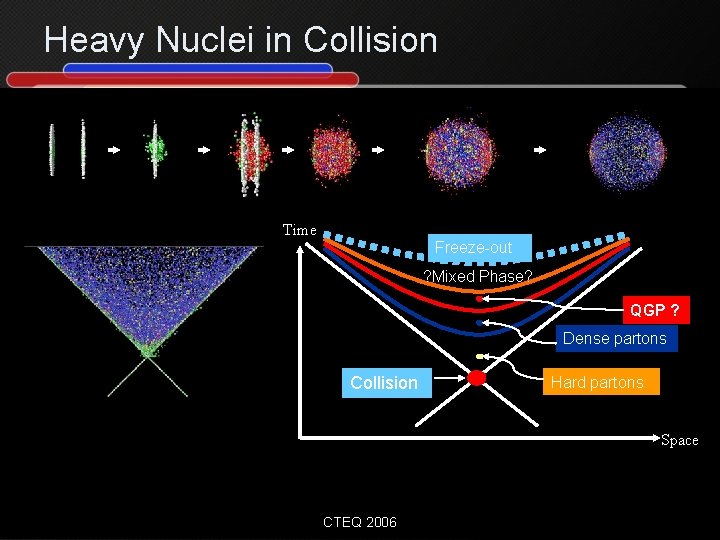
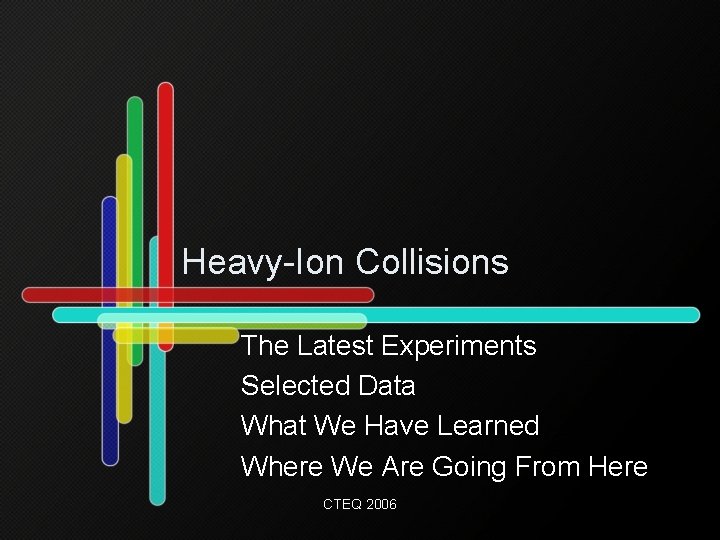
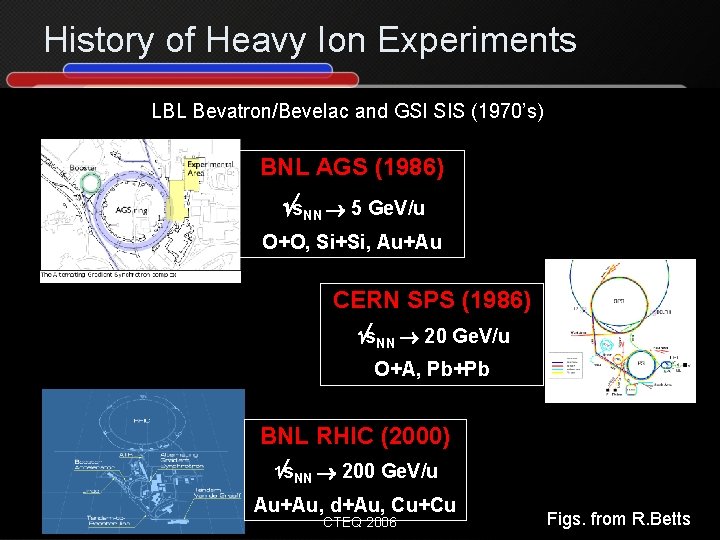
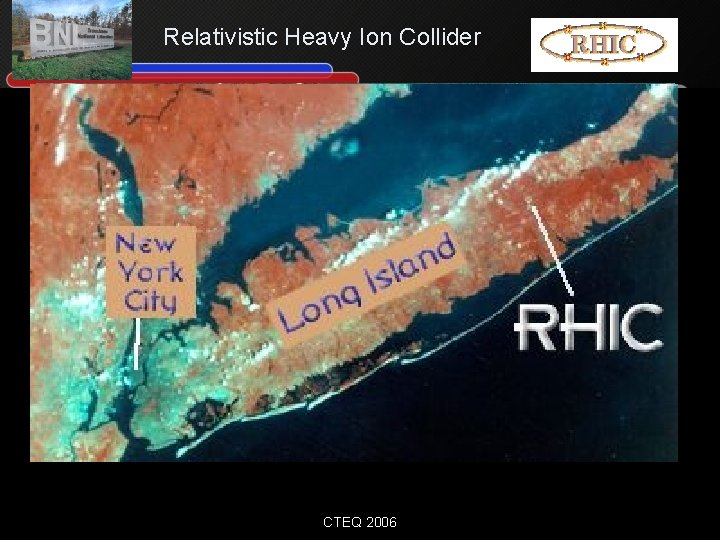
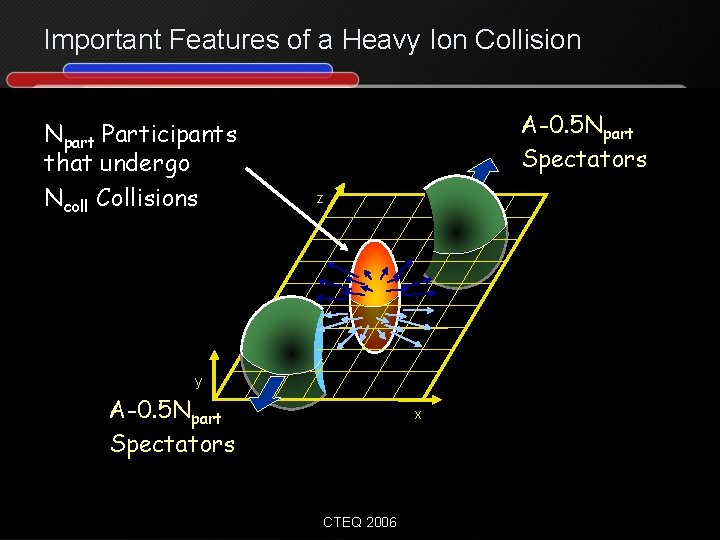
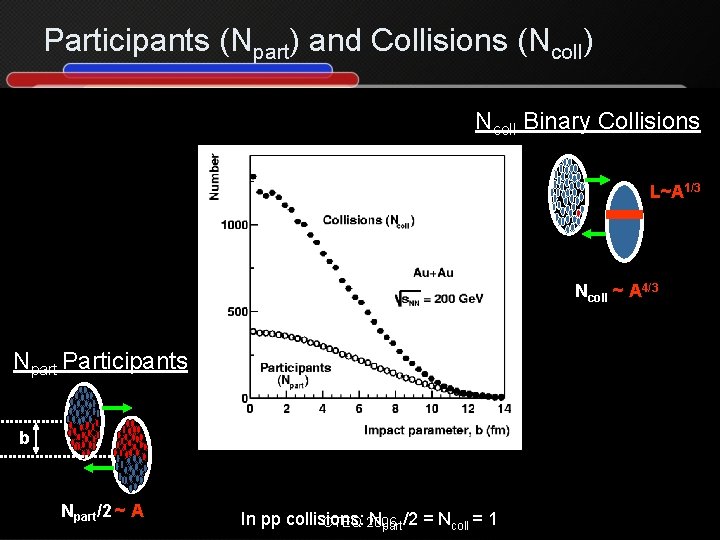
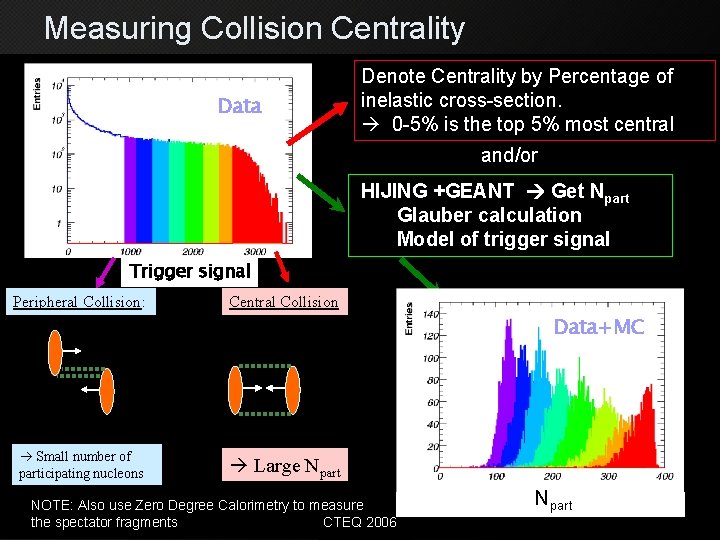
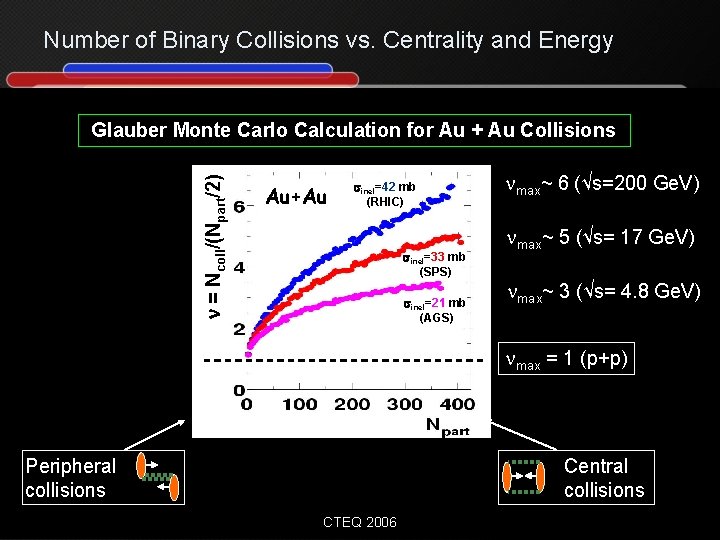
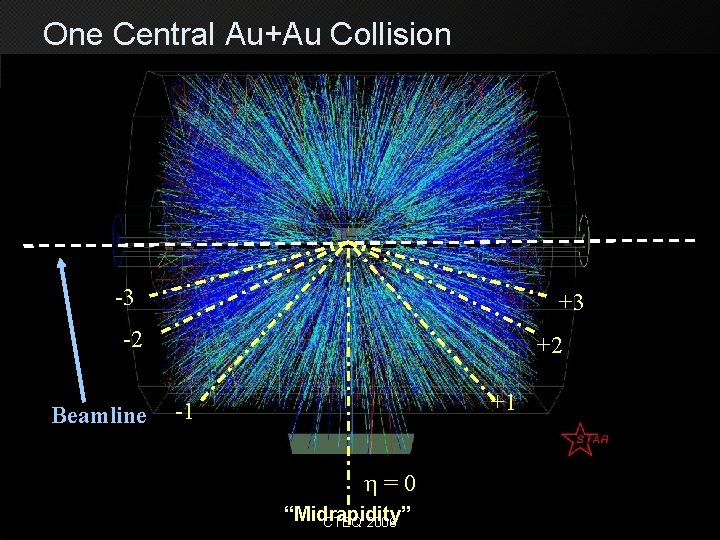
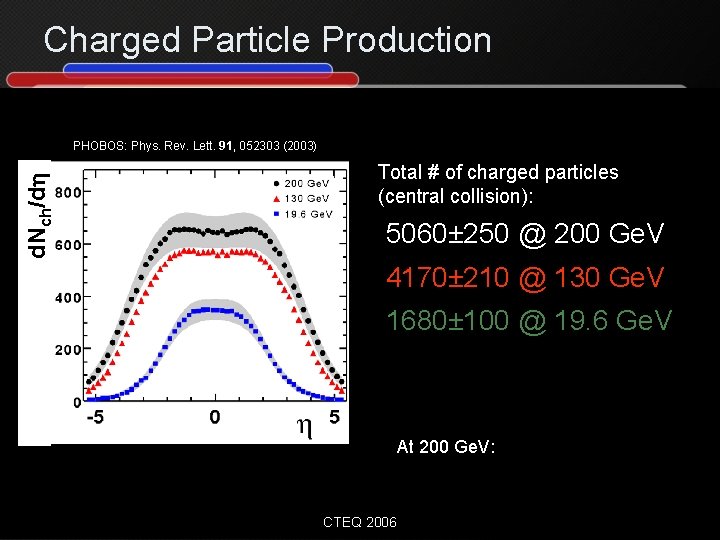
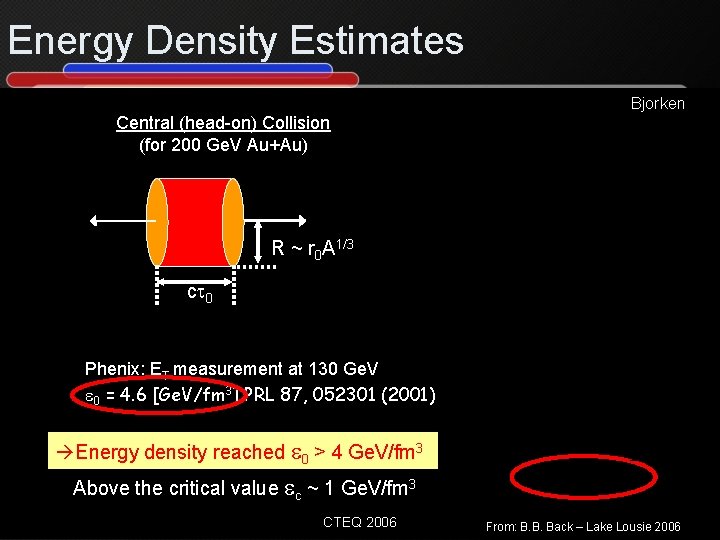
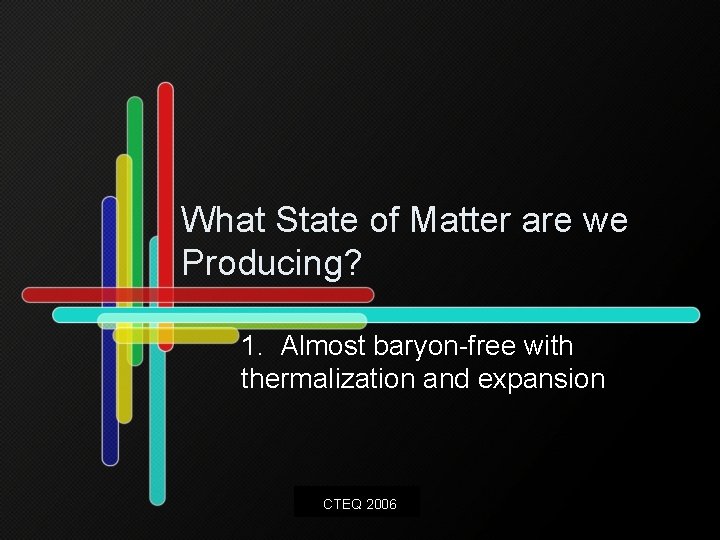
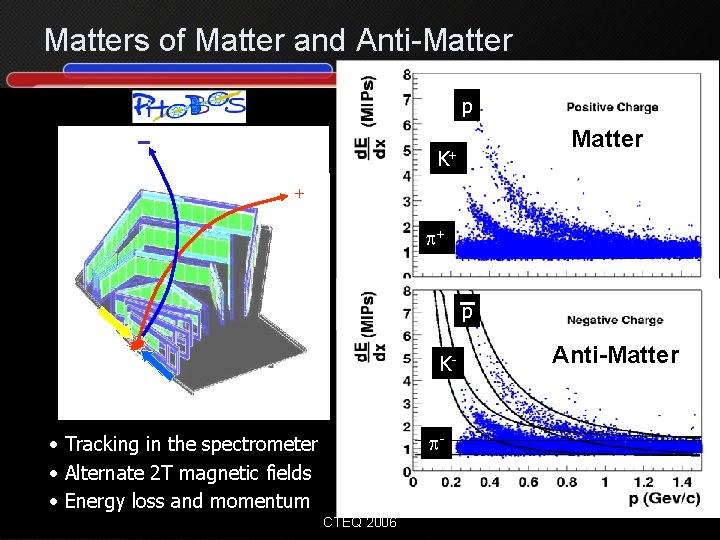
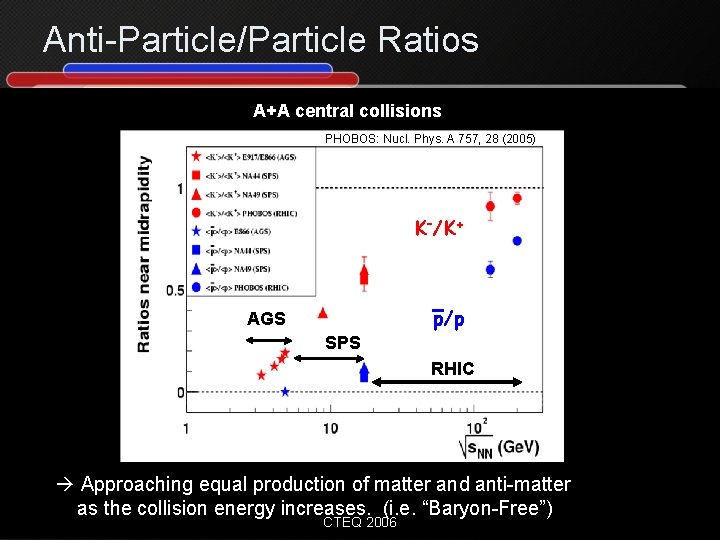
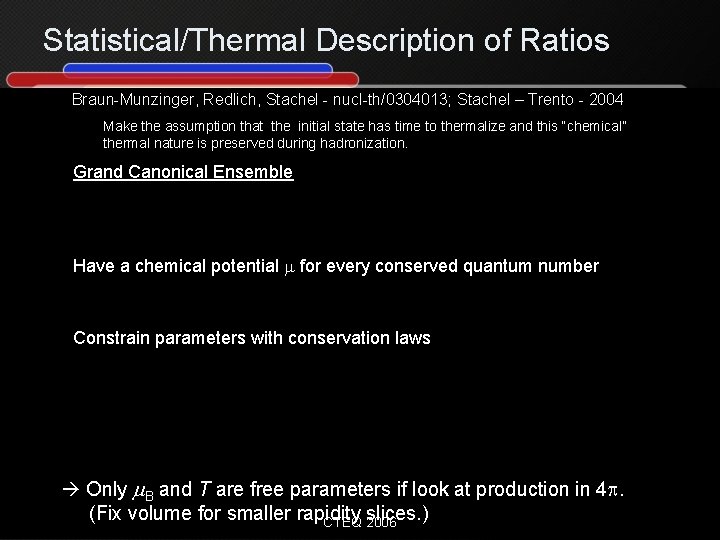
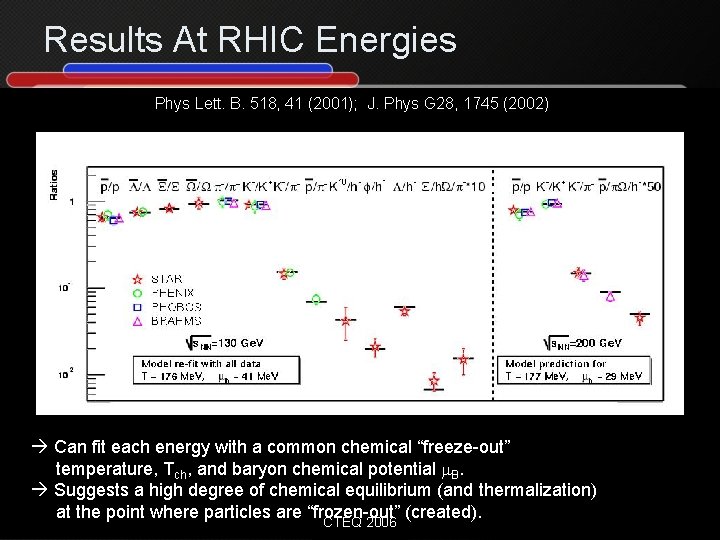
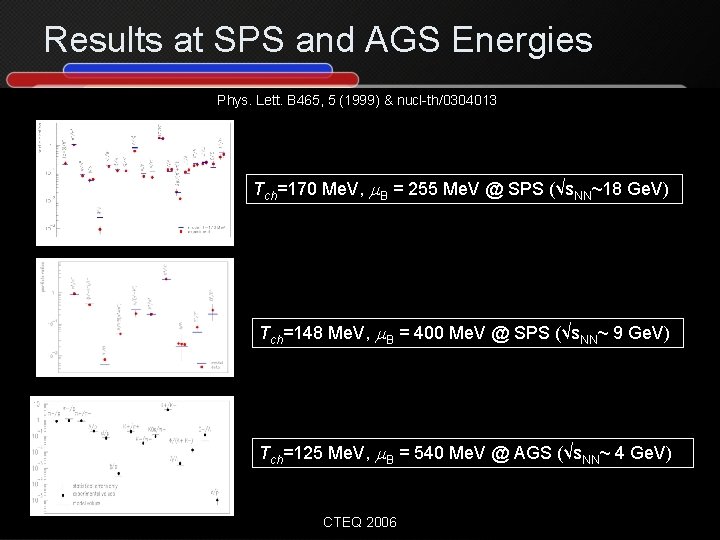
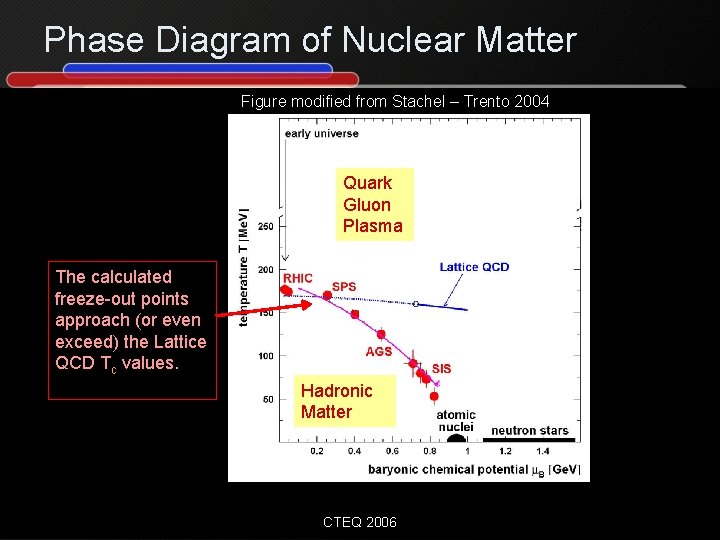
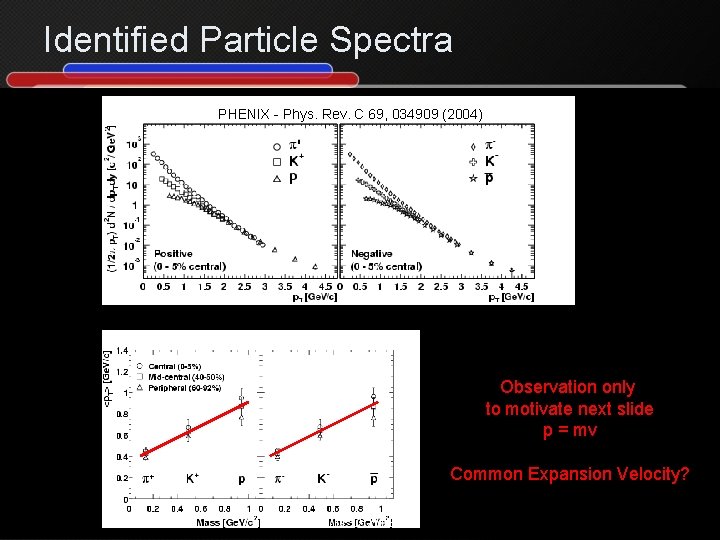
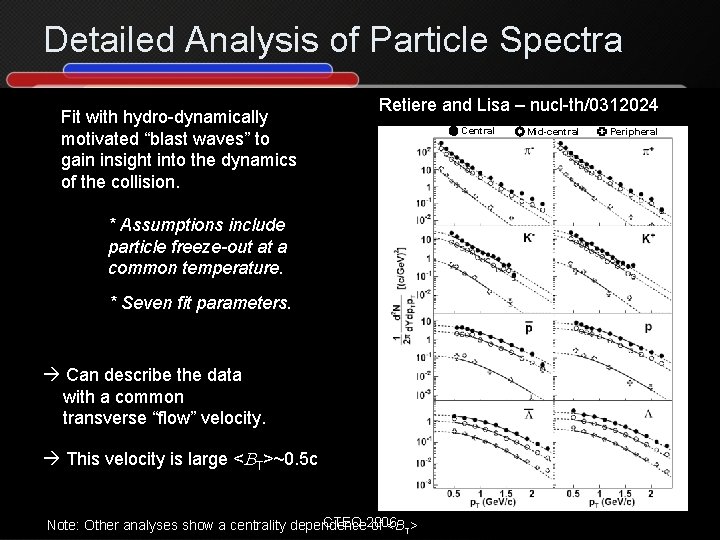
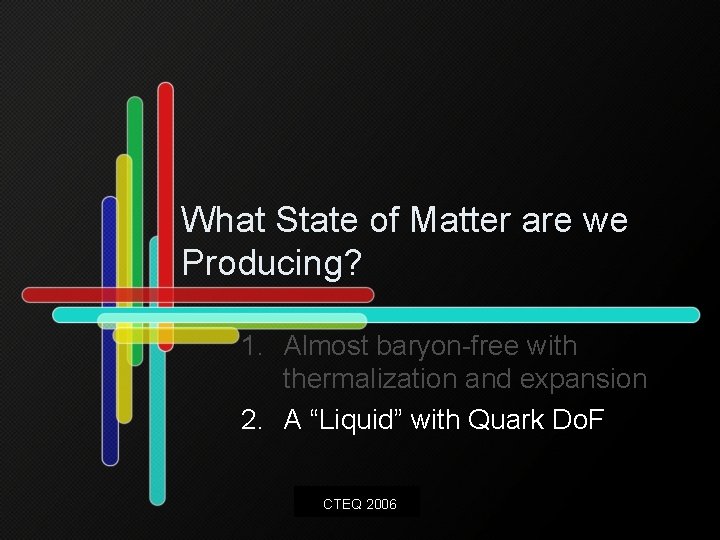
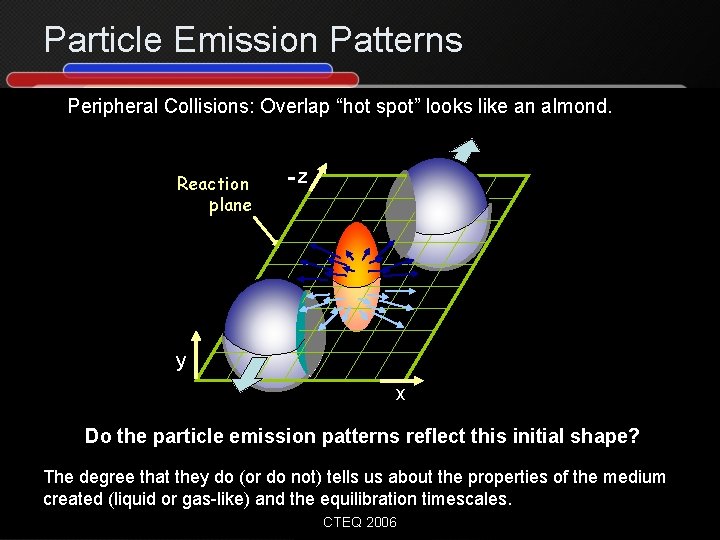
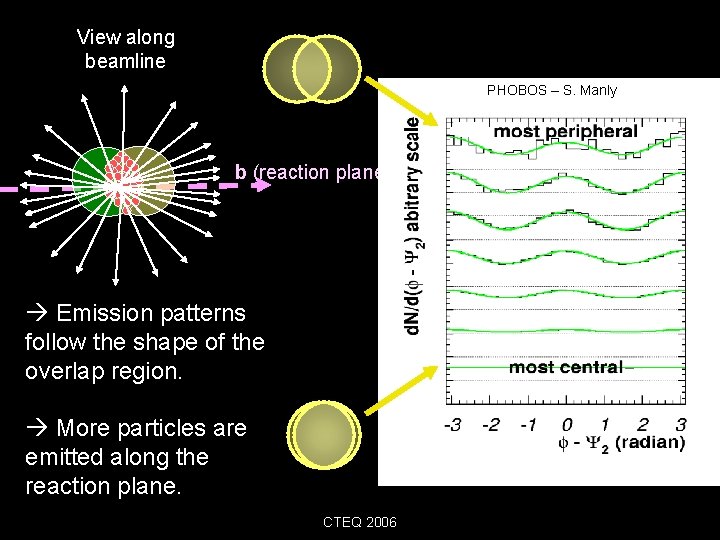
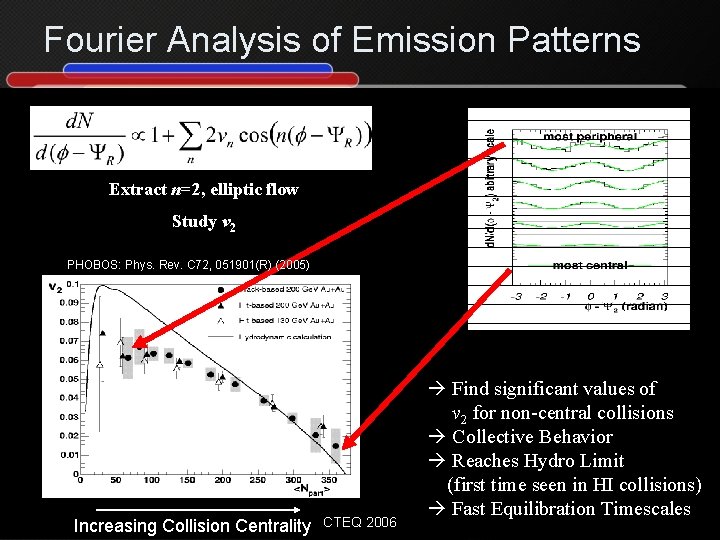
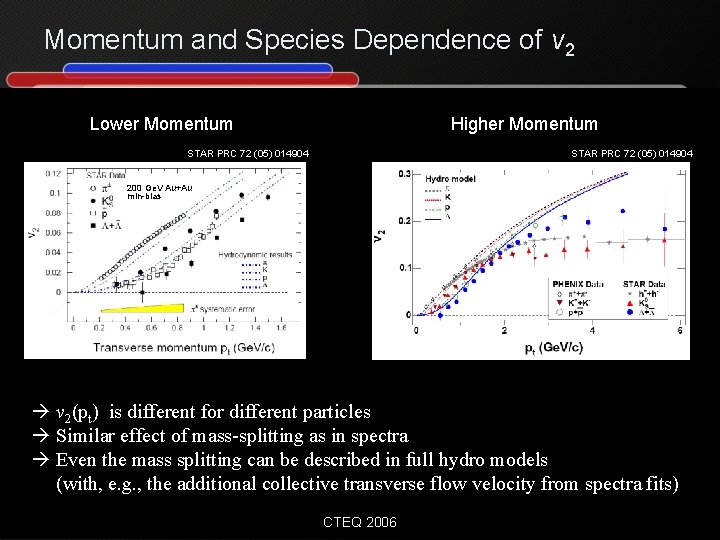
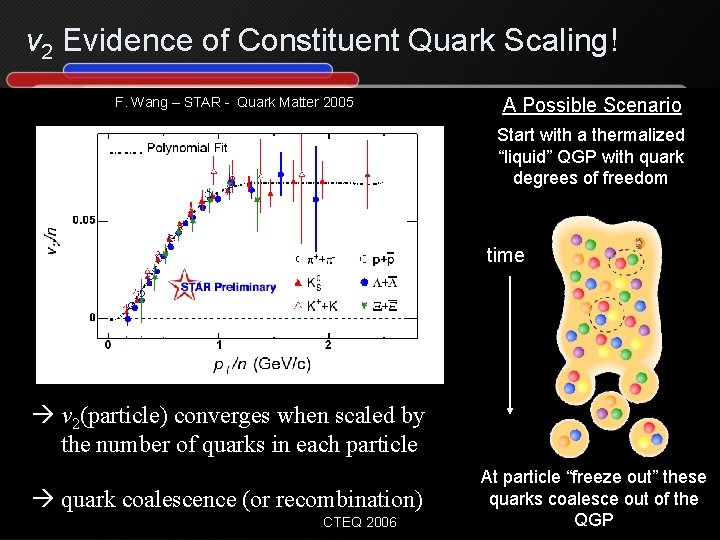
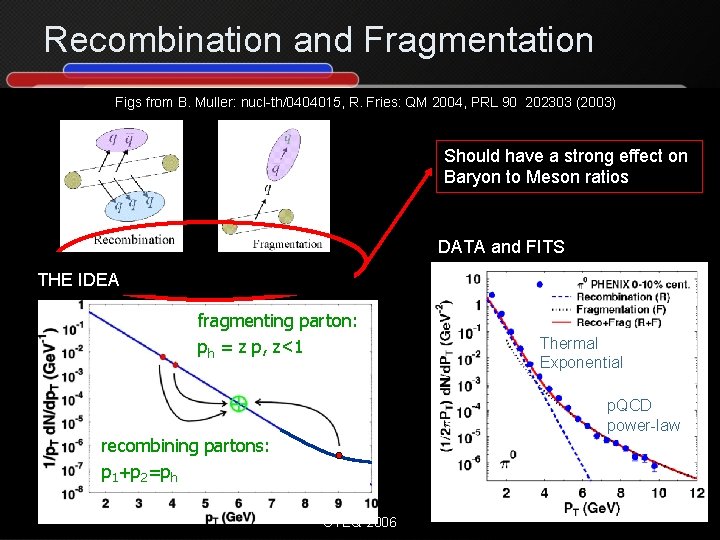
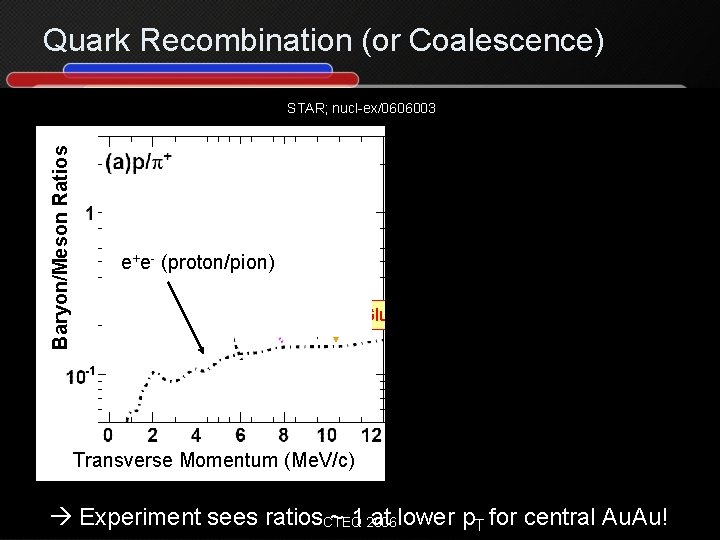
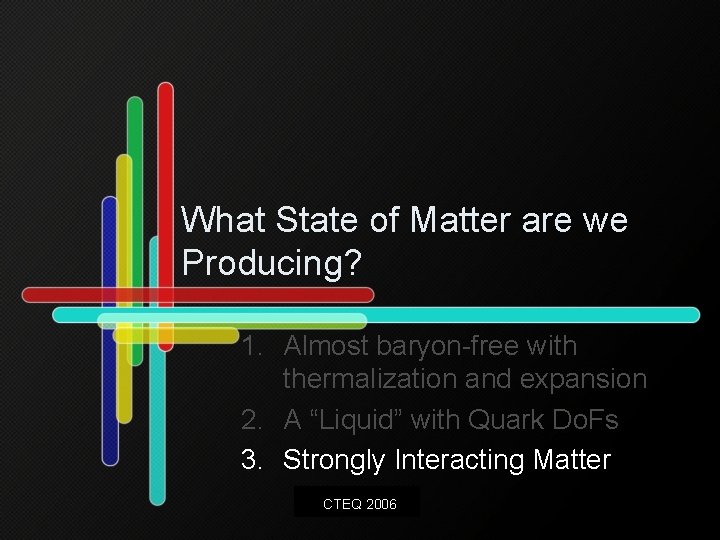
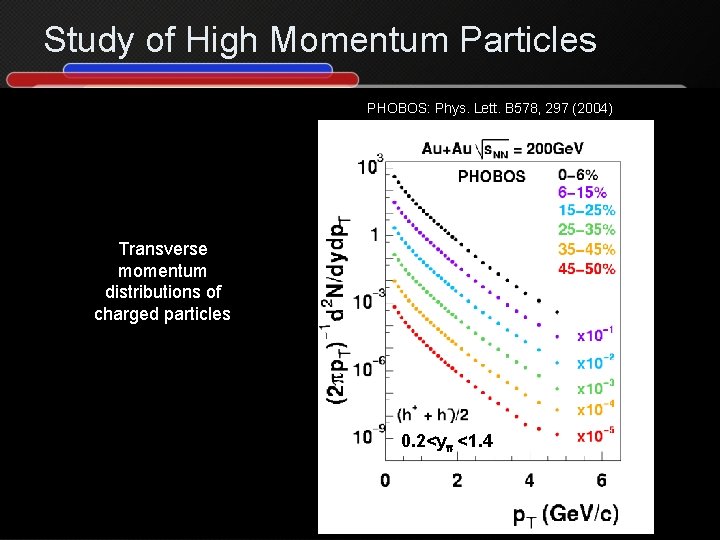
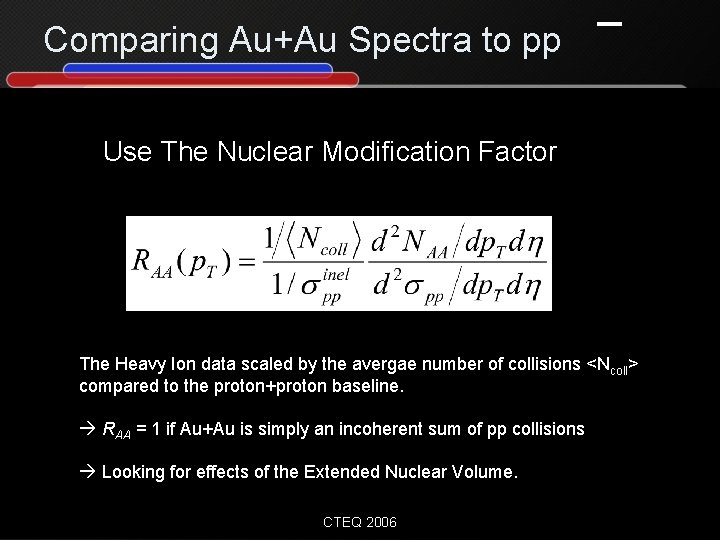
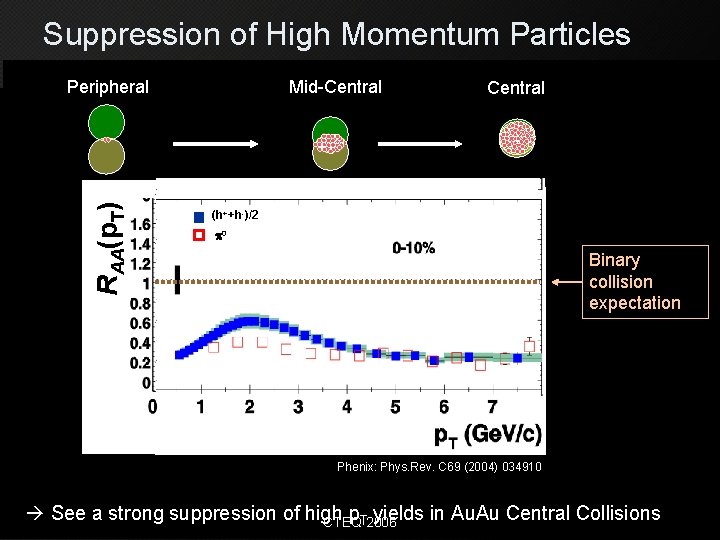
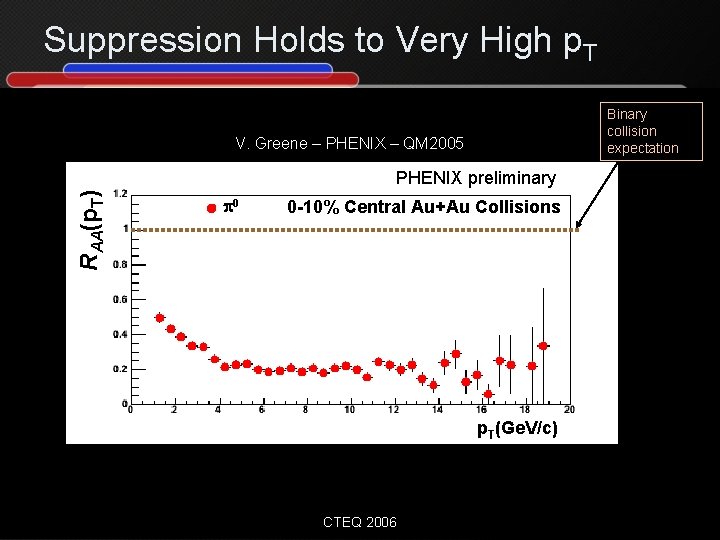
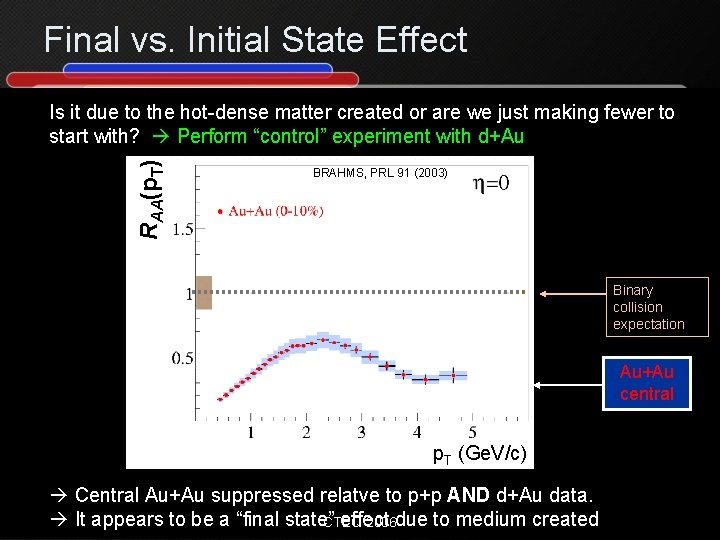
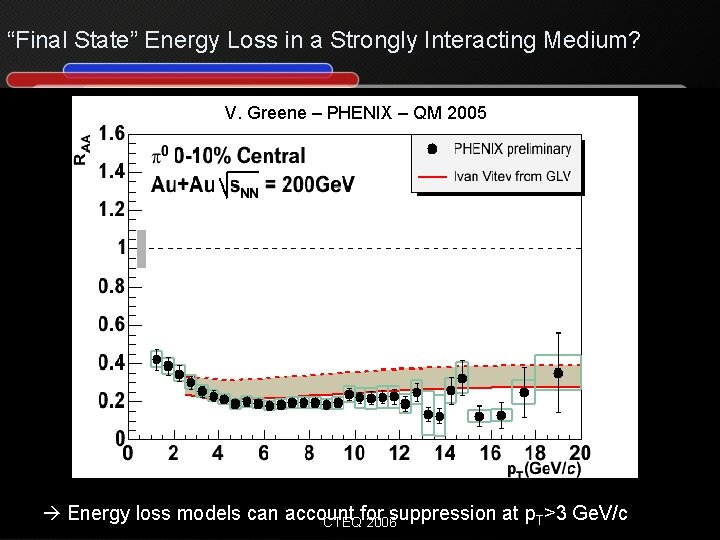
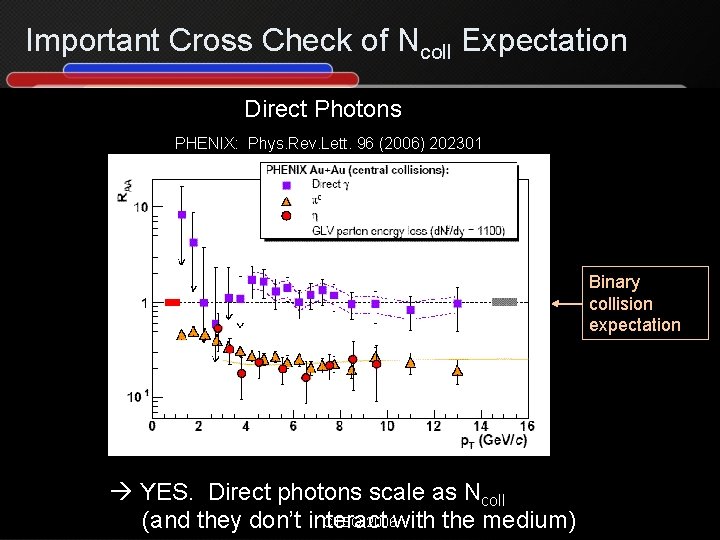
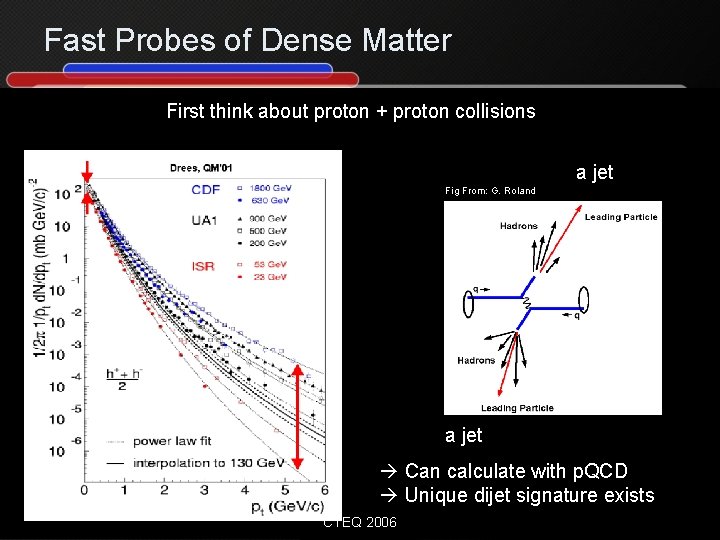
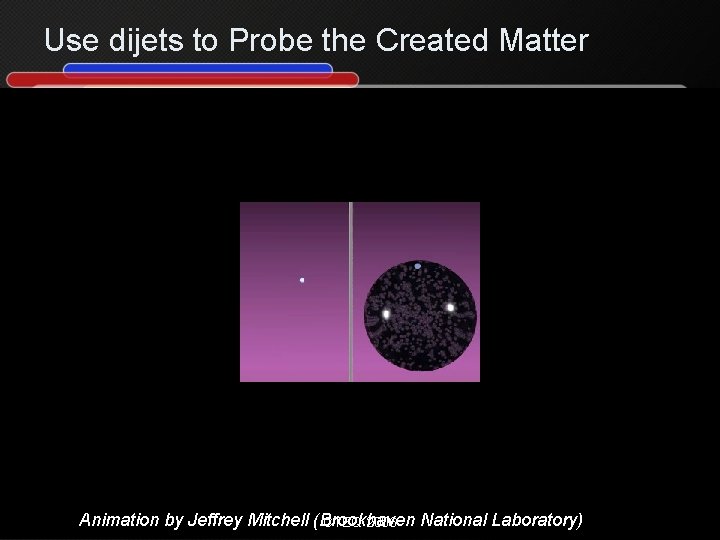
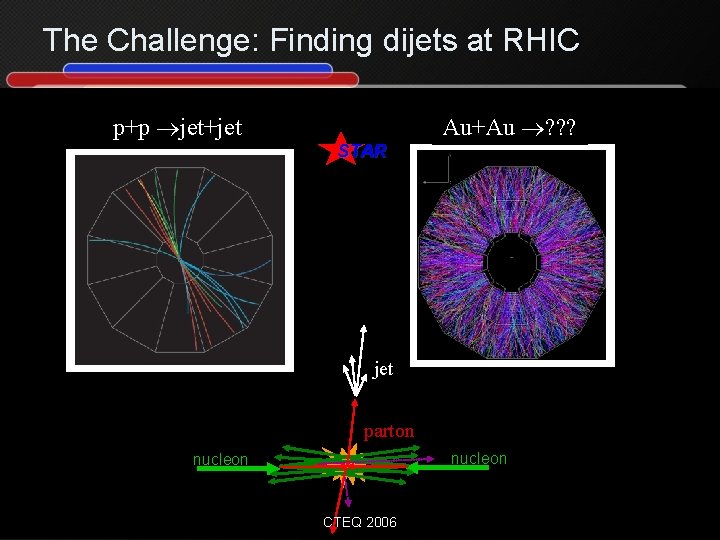
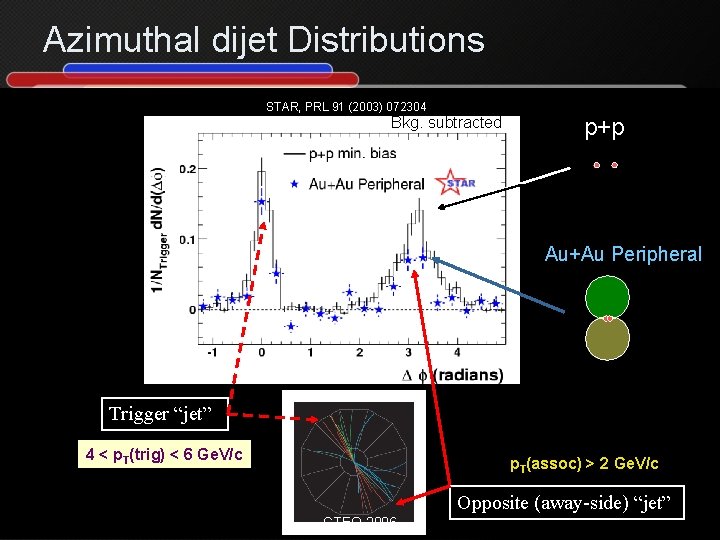
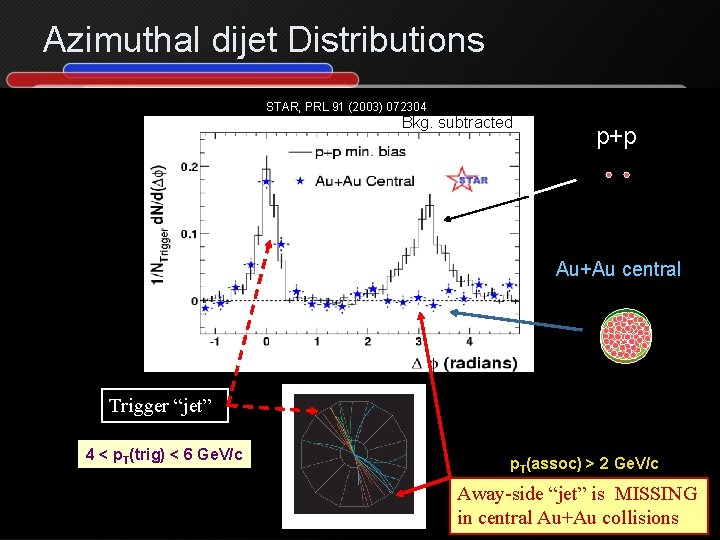
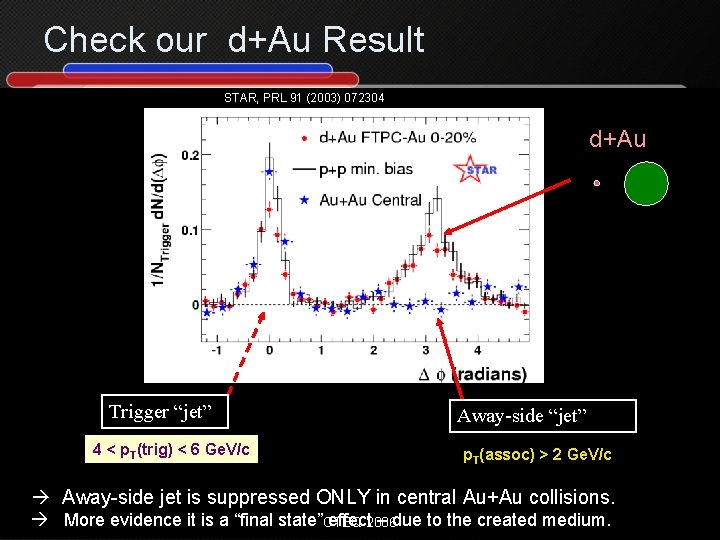
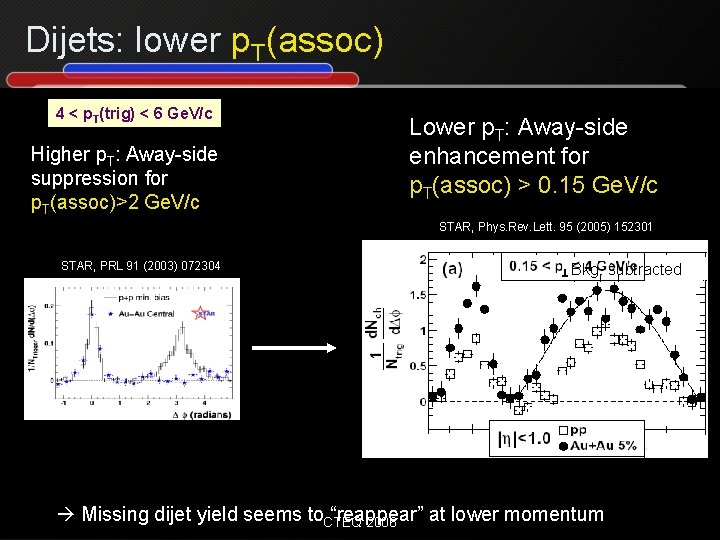
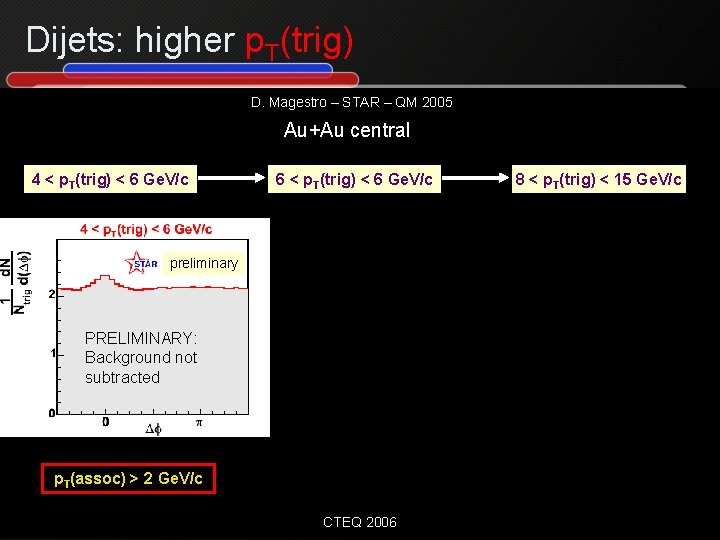
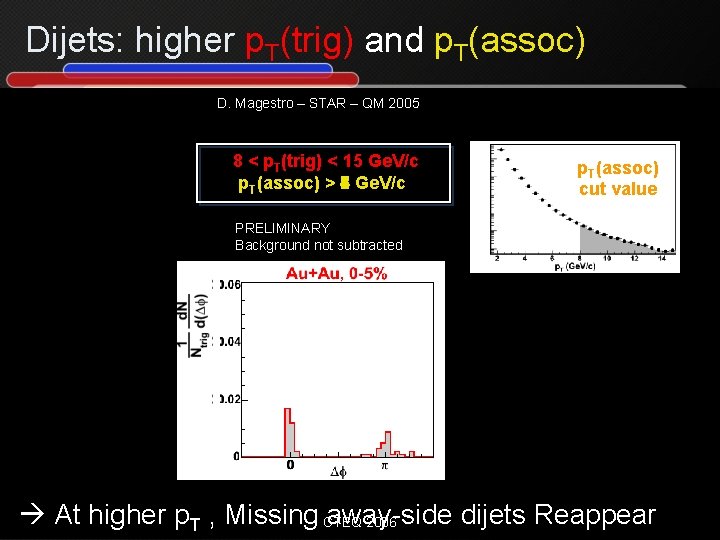
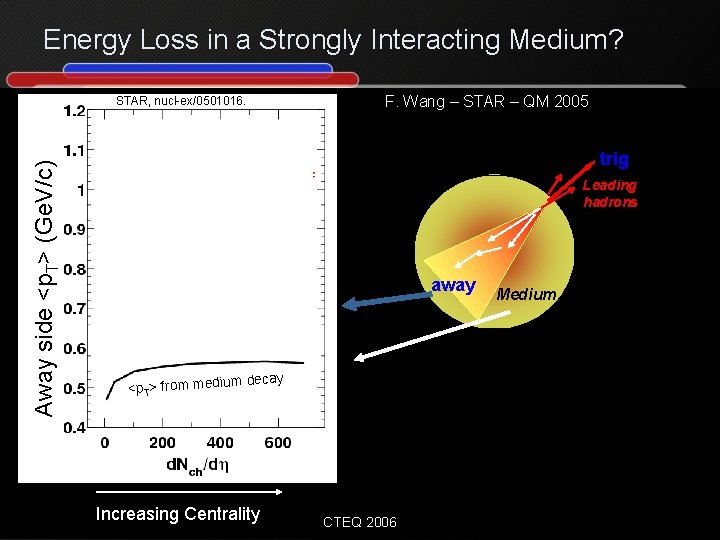
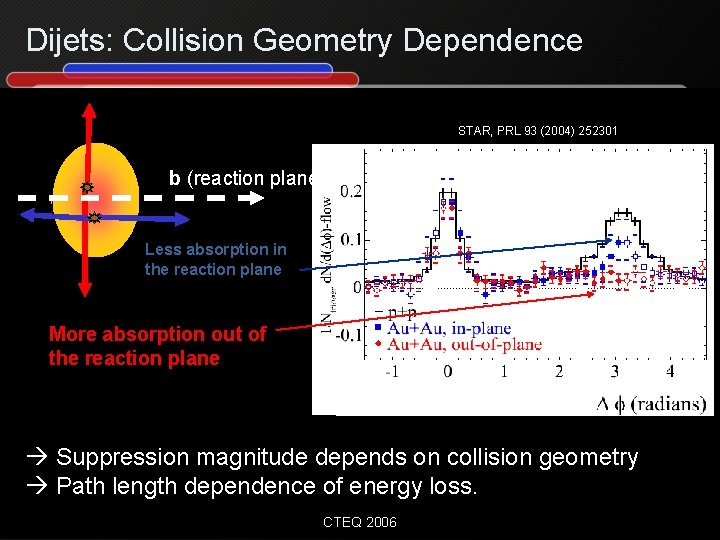
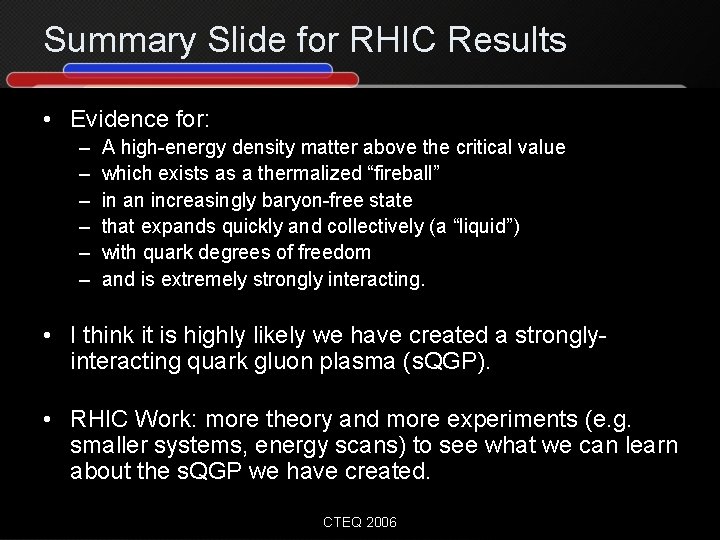
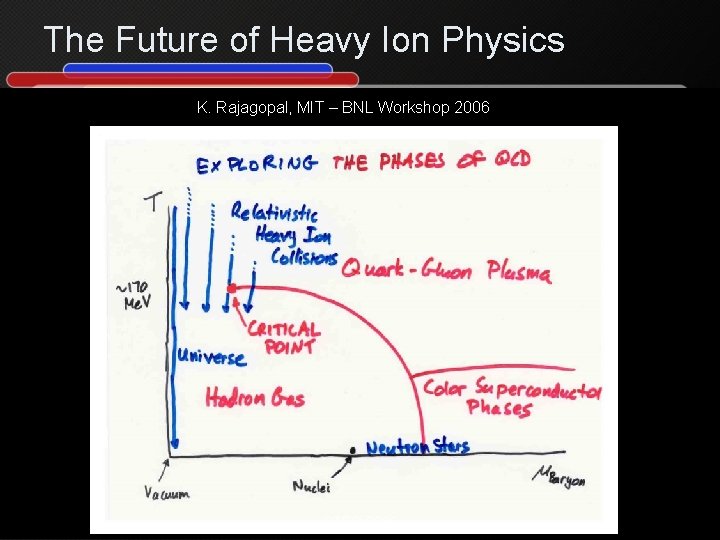
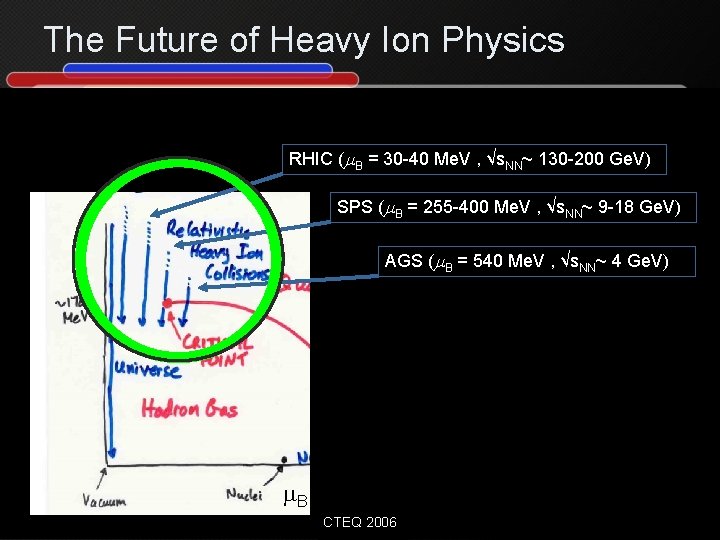
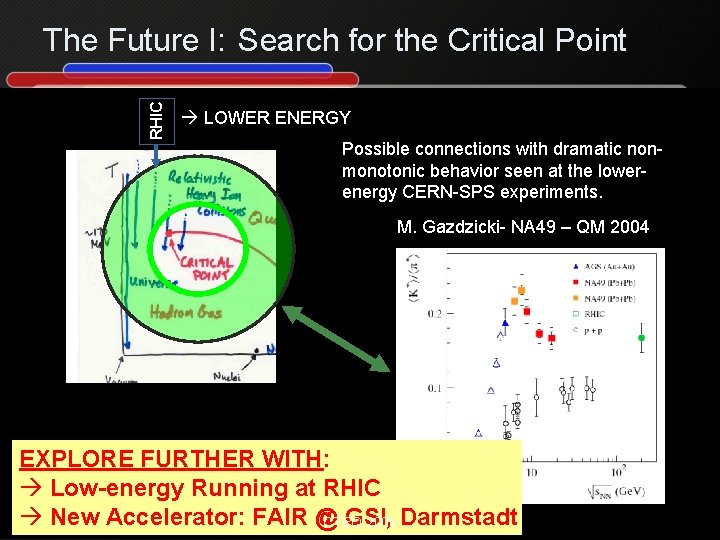
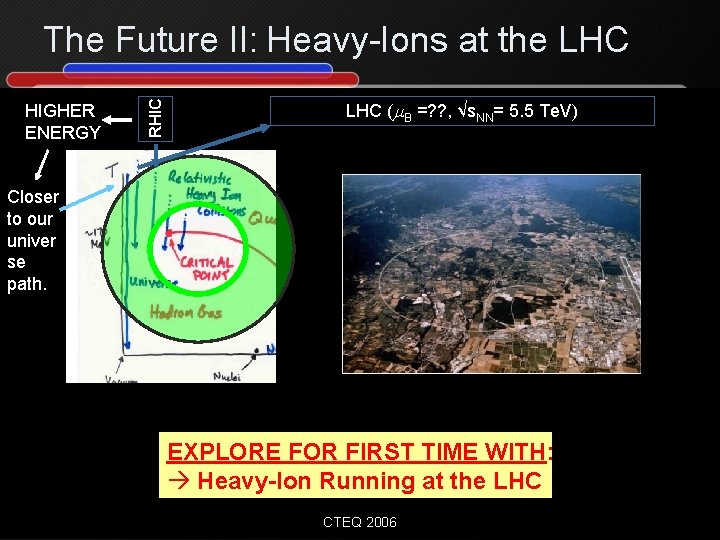
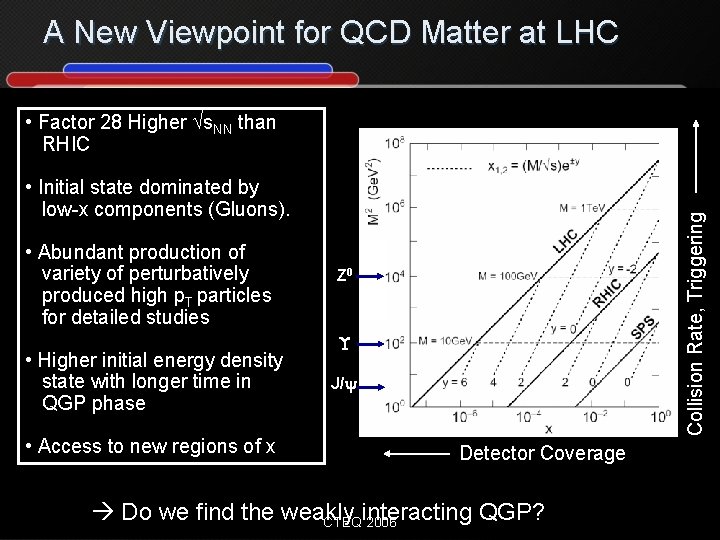
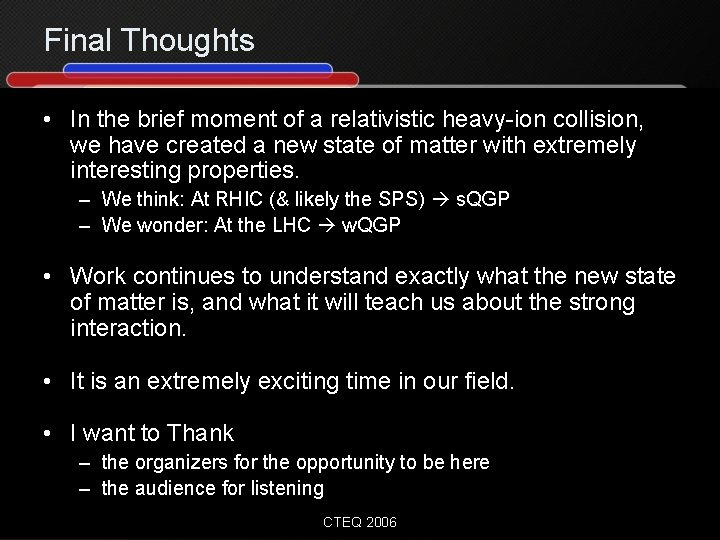
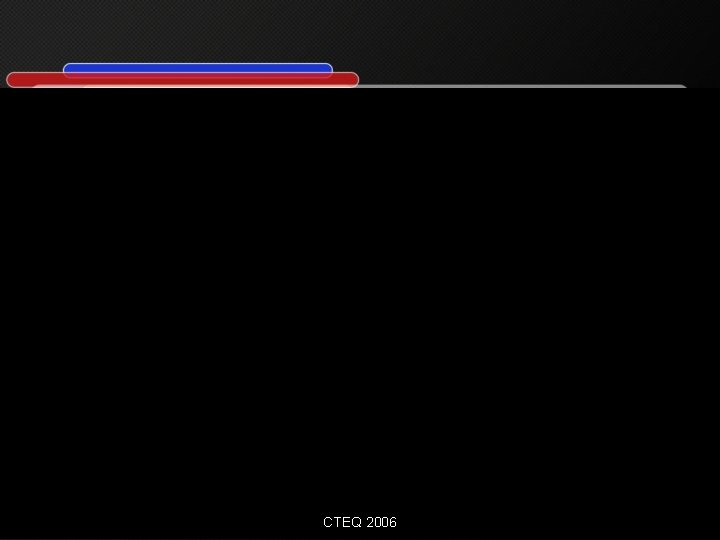
- Slides: 74
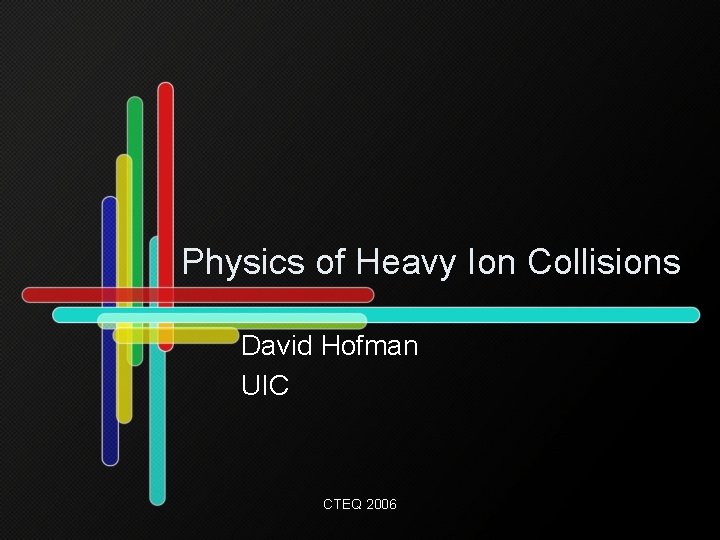
Physics of Heavy Ion Collisions David Hofman UIC CTEQ 2006
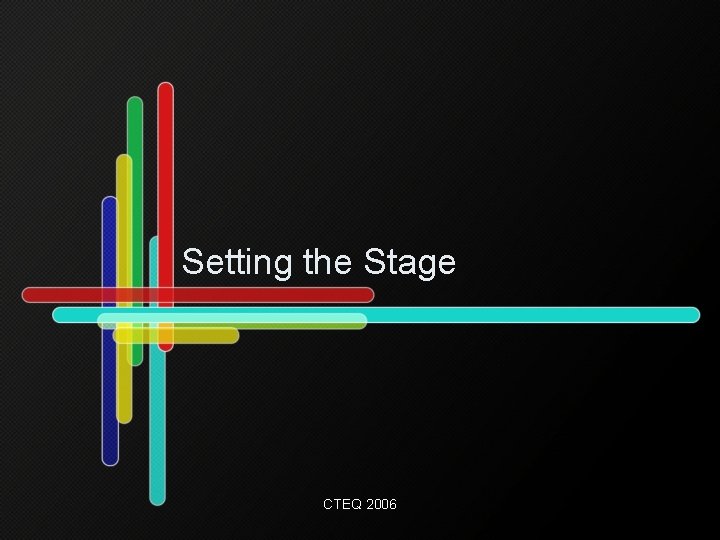
Setting the Stage CTEQ 2006
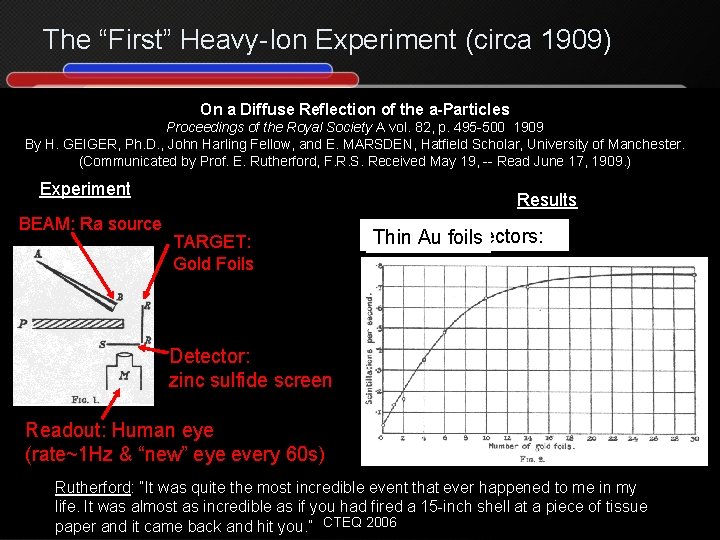
The “First” Heavy-Ion Experiment (circa 1909) On a Diffuse Reflection of the a-Particles Proceedings of the Royal Society A vol. 82, p. 495 -500 1909 By H. GEIGER, Ph. D. , John Harling Fellow, and E. MARSDEN, Hatfield Scholar, University of Manchester. (Communicated by Prof. E. Rutherford, F. R. S. Received May 19, -- Read June 17, 1909. ) Experiment BEAM: Ra source Results TARGET: Gold Foils Detector: zinc sulfide screen Readout: Human eye (rate~1 Hz & “new” eye every 60 s) Different Reflectors: Thin Au foils 62 scintillations/min for Lead 67 “ “ Gold 63 “ “ Platinum 34 “ “ Tin 27 “ “ Silver 14. 5 “ “ Copper 10. 2 “ “ Iron 3. 4 “ “ Aluminum Rutherford: “It was quite the most incredible event that ever happened to me in my life. It was almost as incredible as if you had fired a 15 -inch shell at a piece of tissue paper and it came back and hit you. ” CTEQ 2006
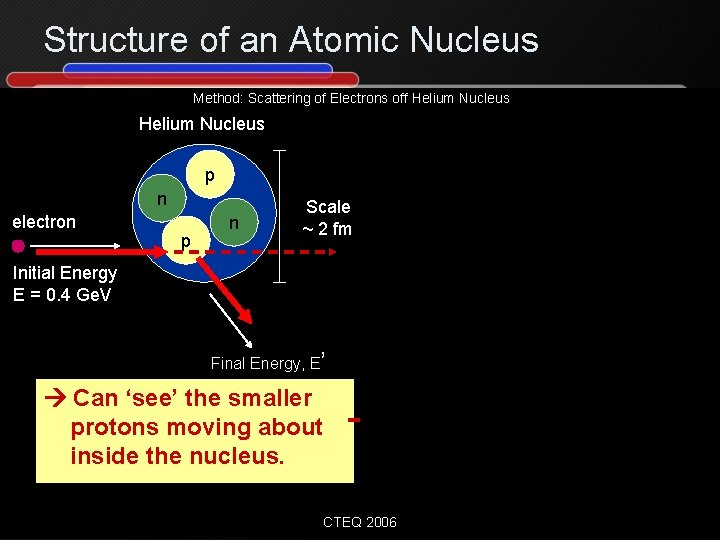
Structure of an Atomic Nucleus Method: Scattering of Electrons off Helium Nucleus From D. H. Perkins, Intro. to High Energy Physics n electron p n Scale ~ 2 fm Initial Energy E = 0. 4 Ge. V Final Energy, E’ Can ‘see’ the smaller protons moving about inside the nucleus. Yield of Electrons (cross section) p CTEQ 2006 Elastic Scattering of Electron off the Nucleus 0. 25 0. 30 0. 35 0. 40 Final Electron Energy, E’ (Ge. V)
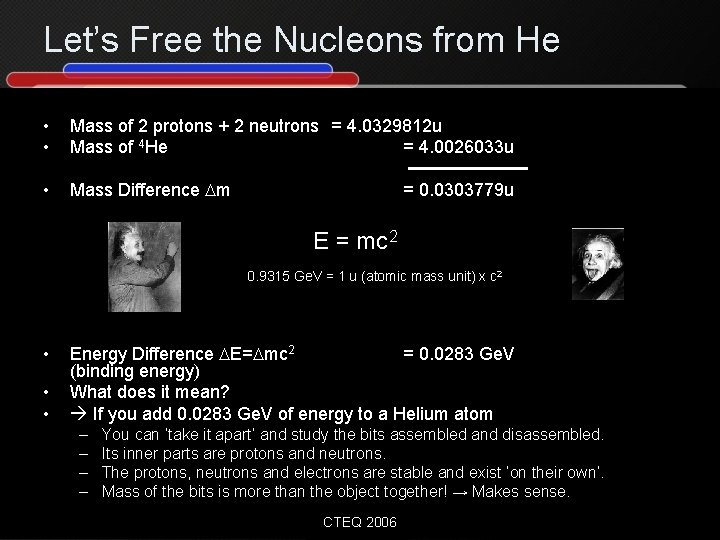
Let’s Free the Nucleons from He • • Mass of 2 protons + 2 neutrons = 4. 0329812 u Mass of 4 He = 4. 0026033 u • Mass Difference Dm = 0. 0303779 u E = mc 2 0. 9315 Ge. V = 1 u (atomic mass unit) x c 2 • • • Energy Difference DE=Dmc 2 = 0. 0283 Ge. V (binding energy) What does it mean? If you add 0. 0283 Ge. V of energy to a Helium atom – – You can ‘take it apart’ and study the bits assembled and disassembled. Its inner parts are protons and neutrons. The protons, neutrons and electrons are stable and exist ‘on their own’. Mass of the bits is more than the object together! → Makes sense. CTEQ 2006
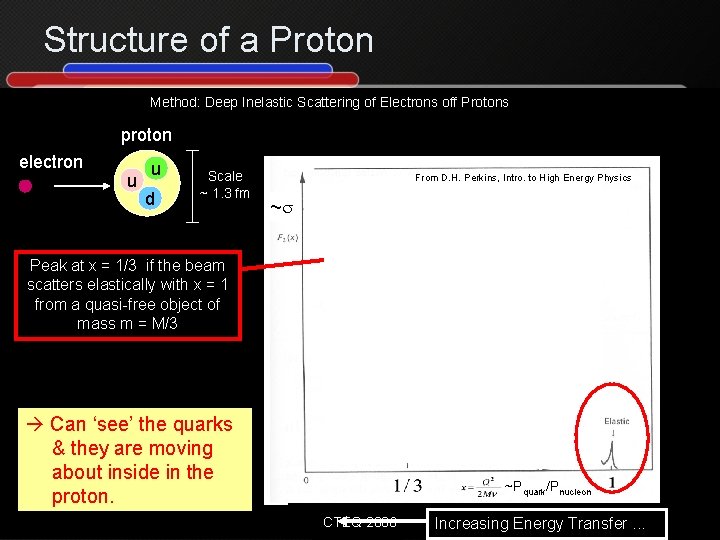
Structure of a Proton Method: Deep Inelastic Scattering of Electrons off Protons proton electron u u d Scale ~ 1. 3 fm From D. H. Perkins, Intro. to High Energy Physics ~s Peak at x = 1/3 if the beam scatters elastically with x = 1 from a quasi-free object of mass m = M/3 Can ‘see’ the quarks & they are moving about inside in the proton. ~Pquark/Pnucleon CTEQ 2006 Increasing Energy Transfer. . .
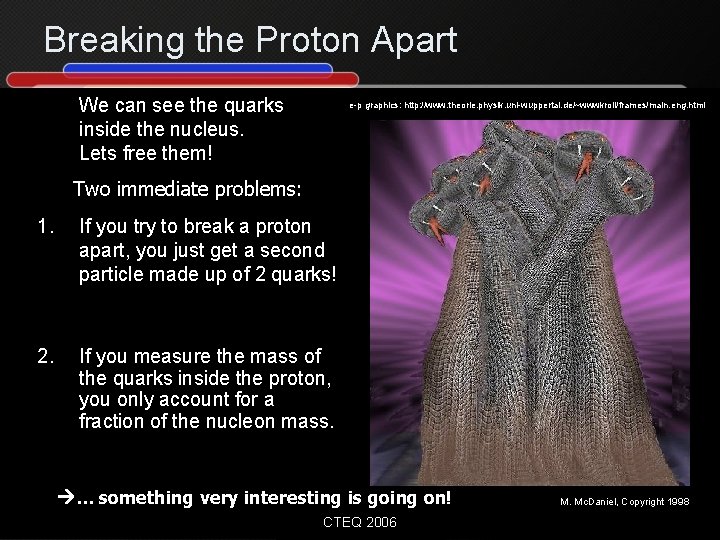
Breaking the Proton Apart We can see the quarks inside the nucleus. Lets free them! e-p graphics: http: //www. theorie. physik. uni-wuppertal. de/~wwwkroll/frames/main. eng. html Electron Two immediate problems: 1. If you try to break a proton apart, you just get a second particle made up of 2 quarks! Electron 2. If you measure the mass of the quarks inside the proton, you only account for a fraction of the nucleon mass. … something very interesting is going on! CTEQ 2006 M. Mc. Daniel, Copyright 1998
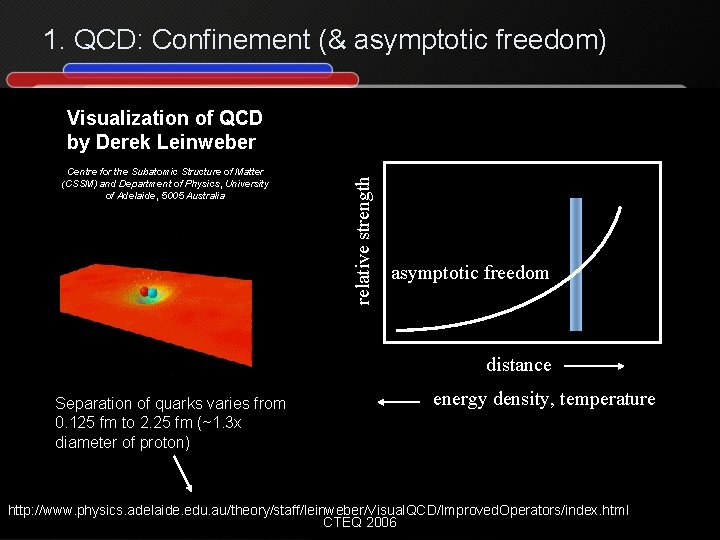
1. QCD: Confinement (& asymptotic freedom) Centre for the Subatomic Structure of Matter (CSSM) and Department of Physics, University of Adelaide, 5005 Australia relative strength Visualization of QCD by Derek Leinweber asymptotic freedom distance Separation of quarks varies from 0. 125 fm to 2. 25 fm (~1. 3 x diameter of proton) energy density, temperature http: //www. physics. adelaide. edu. au/theory/staff/leinweber/Visual. QCD/Improved. Operators/index. html CTEQ 2006
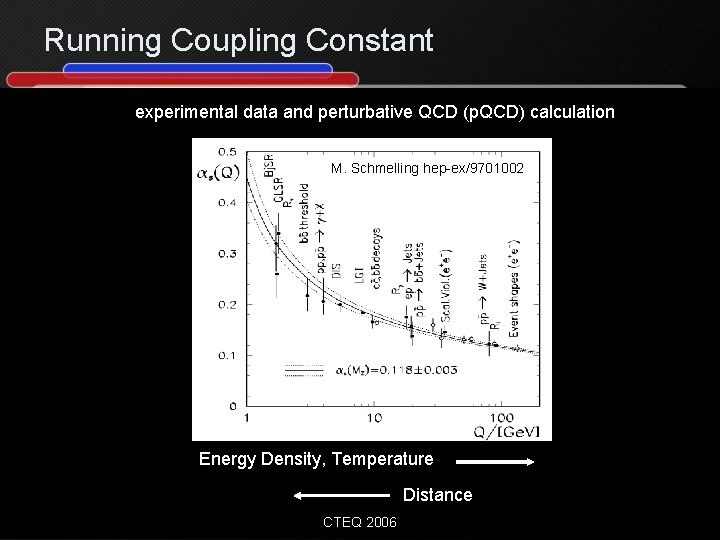
Running Coupling Constant experimental data and perturbative QCD (p. QCD) calculation M. Schmelling hep-ex/9701002 Energy Density, Temperature Distance CTEQ 2006
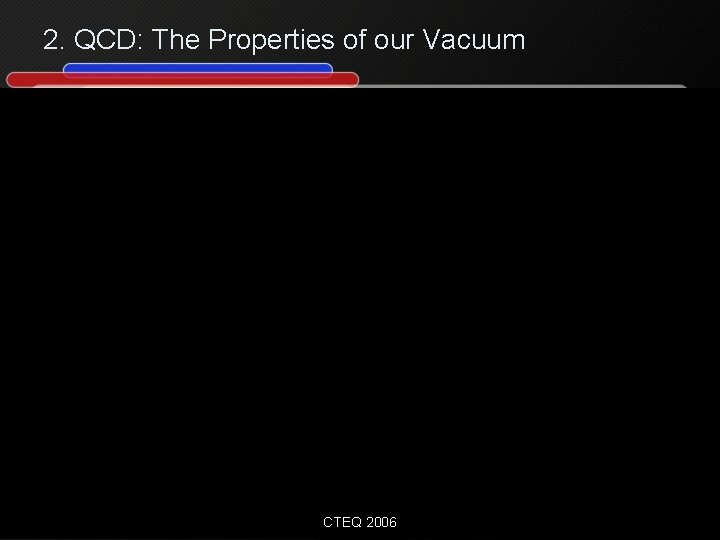
2. QCD: The Properties of our Vacuum Visualization of QCD: by Derek Leinweber (same references as earlier slide) Action Density (~Energy Density) Topological Charge Density (measure of winding of gluon field lines) Volume 2. 4 x 3. 6 fm 3 (Can hold a couple of protons) “Contrary to the concept of an empty vacuum, QCD induces chromoelectric and chromo-magnetic fields throughout space-time in its lowest energy state. ” - D. Leinweber CTEQ 2006
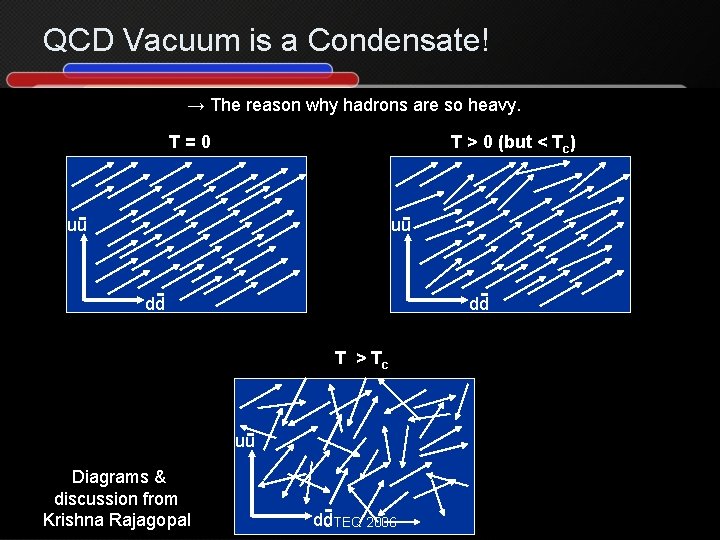
QCD Vacuum is a Condensate! → The reason why hadrons are so heavy. T=0 T > 0 (but < Tc) uu uu dd dd T > Tc uu Diagrams & discussion from Krishna Rajagopal dd CTEQ 2006
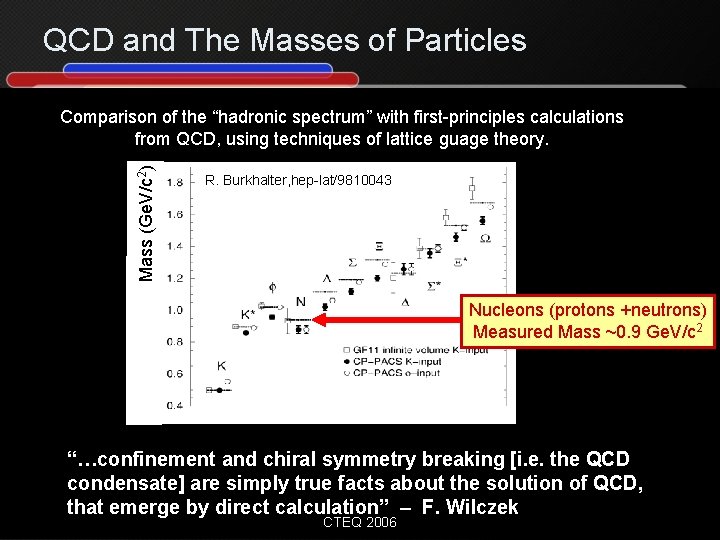
QCD and The Masses of Particles Mass (Ge. V/c 2) Comparison of the “hadronic spectrum” with first-principles calculations from QCD, using techniques of lattice guage theory. R. Burkhalter, hep-lat/9810043 Nucleons (protons +neutrons) Measured Mass ~0. 9 Ge. V/c 2 “…confinement and chiral symmetry breaking [i. e. the QCD condensate] are simply true facts about the solution of QCD, that emerge by direct calculation” – F. Wilczek CTEQ 2006
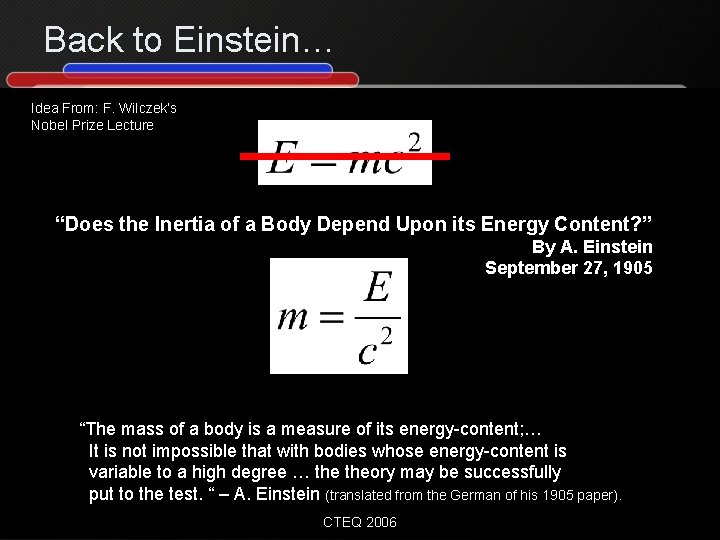
Back to Einstein… Idea From: F. Wilczek’s Nobel Prize Lecture “Does the Inertia of a Body Depend Upon its Energy Content? ” By A. Einstein September 27, 1905 “The mass of a body is a measure of its energy-content; … It is not impossible that with bodies whose energy-content is variable to a high degree … theory may be successfully put to the test. “ – A. Einstein (translated from the German of his 1905 paper). CTEQ 2006
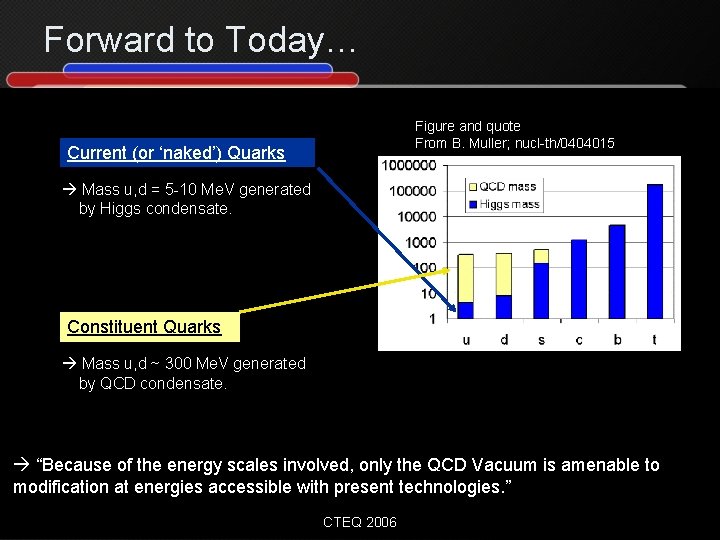
Forward to Today… Figure and quote From B. Muller; nucl-th/0404015 Current (or ‘naked’) Quarks Mass u, d = 5 -10 Me. V generated by Higgs condensate. Constituent Quarks Mass u, d ~ 300 Me. V generated by QCD condensate. “Because of the energy scales involved, only the QCD Vacuum is amenable to modification at energies accessible with present technologies. ” CTEQ 2006
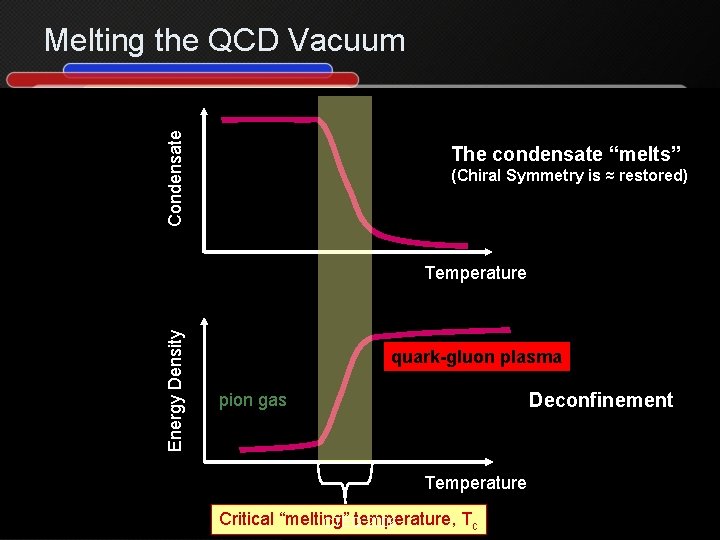
Condensate Melting the QCD Vacuum The condensate “melts” (Chiral Symmetry is ≈ restored) Energy Density Temperature quark-gluon plasma Deconfinement pion gas Temperature Critical “melting” temperature, Tc CTEQ 2006
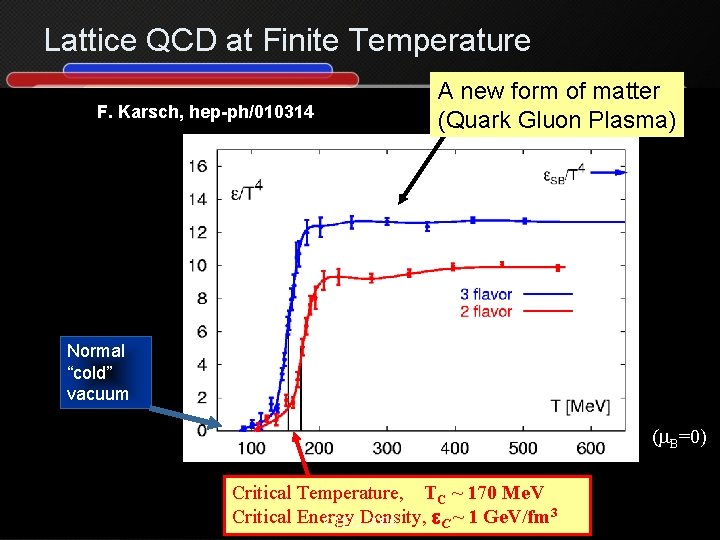
Lattice QCD at Finite Temperature F. Karsch, hep-ph/010314 A new form of matter (Quark Gluon Plasma) Normal “cold” vacuum (m. B=0) Critical Temperature, TC ~ 170 Me. V Critical Energy e. C ~ 1 Ge. V/fm 3 CTEQDensity, 2006
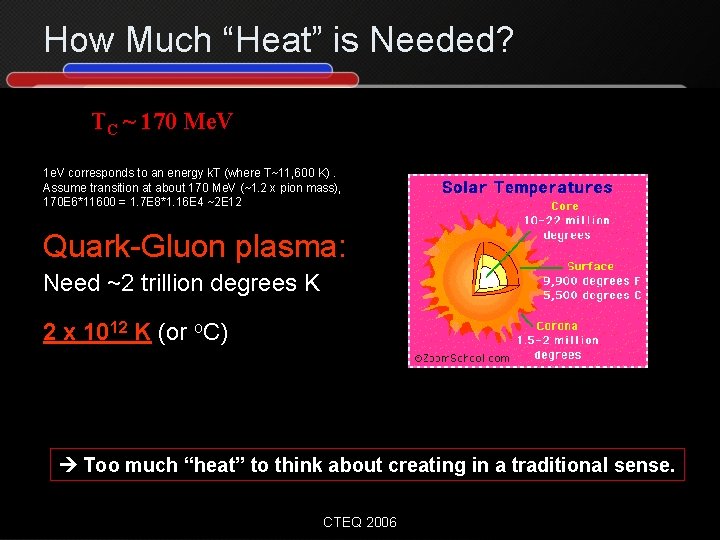
How Much “Heat” is Needed? TC ~ 170 Me. V 1 e. V corresponds to an energy k. T (where T~11, 600 K). Assume transition at about 170 Me. V (~1. 2 x pion mass), 170 E 6*11600 = 1. 7 E 8*1. 16 E 4 ~2 E 12 Quark-Gluon plasma: Need ~2 trillion degrees K 2 x 1012 K (or o. C) Too much “heat” to think about creating in a traditional sense. CTEQ 2006
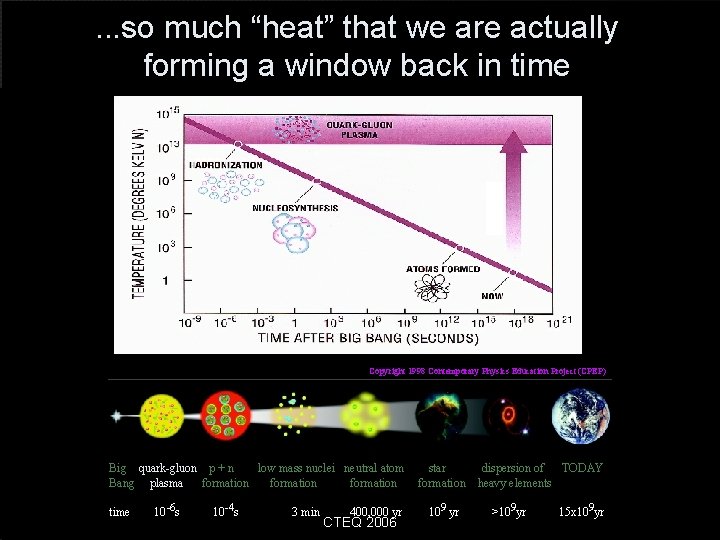
. . . so much “heat” that we are actually forming a window back in time Copyright 1998 Contemporary Physics Education Project (CPEP) Big quark-gluon p + n low mass nuclei neutral atom Bang plasma formation time 10 -6 s 10 -4 s 3 min 400, 000 yr CTEQ 2006 star formation 109 yr dispersion of TODAY heavy elements >109 yr 15 x 109 yr
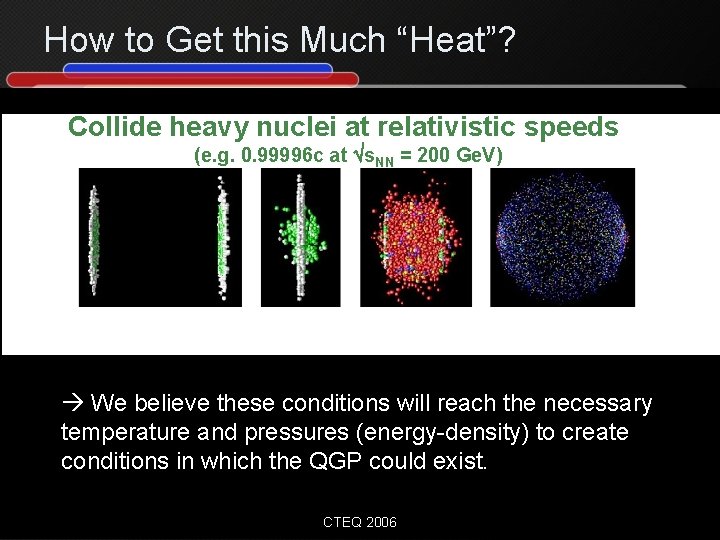
How to Get this Much “Heat”? Collide heavy nuclei at relativistic speeds (e. g. 0. 99996 c at s. NN = 200 Ge. V) We believe these conditions will reach the necessary temperature and pressures (energy-density) to create conditions in which the QGP could exist. CTEQ 2006
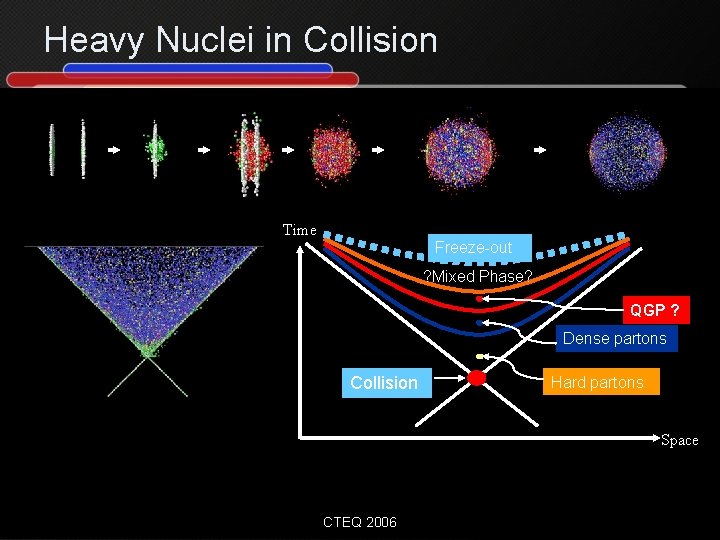
Heavy Nuclei in Collision Time Freeze-out ? Mixed Phase? QGP ? Dense partons Collision Hard partons Space CTEQ 2006
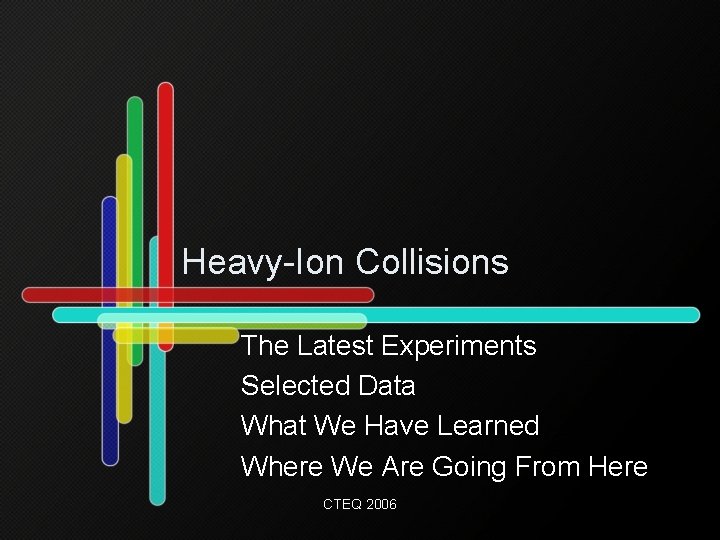
Heavy-Ion Collisions The Latest Experiments Selected Data What We Have Learned Where We Are Going From Here CTEQ 2006
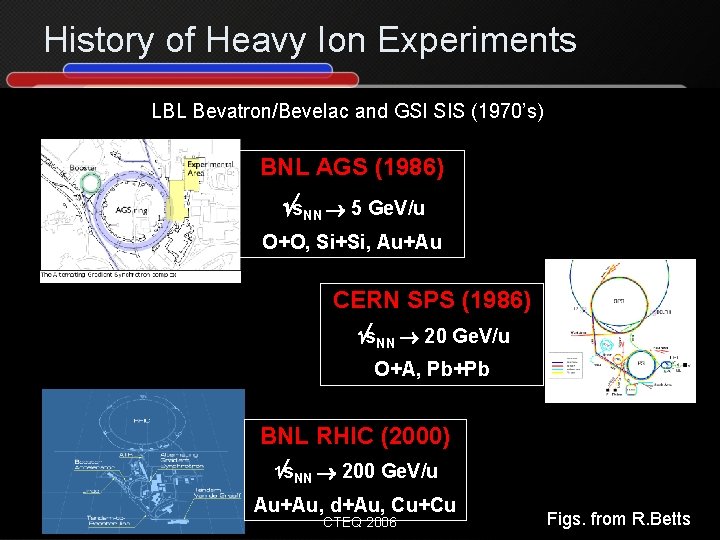
History of Heavy Ion Experiments LBL Bevatron/Bevelac and GSI SIS (1970’s) BNL AGS (1986) s. NN 5 Ge. V/u O+O, Si+Si, Au+Au CERN SPS (1986) s. NN 20 Ge. V/u O+A, Pb+Pb BNL RHIC (2000) s. NN 200 Ge. V/u Au+Au, d+Au, Cu+Cu CTEQ 2006 Figs. from R. Betts
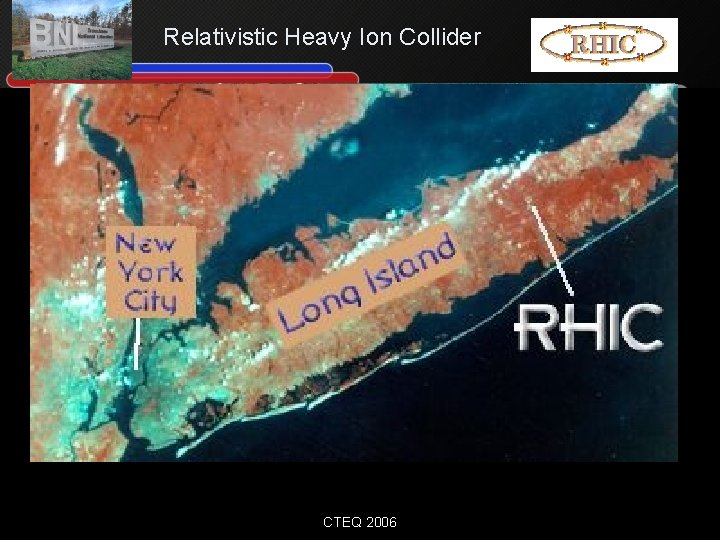
Relativistic Heavy Ion Collider CTEQ 2006
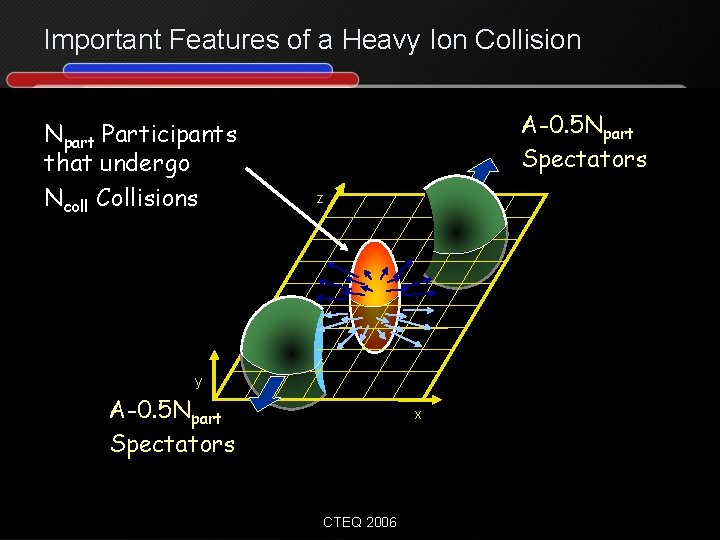
Important Features of a Heavy Ion Collision Npart Participants that undergo Ncoll Collisions A-0. 5 Npart Spectators z y A-0. 5 Npart Spectators x CTEQ 2006
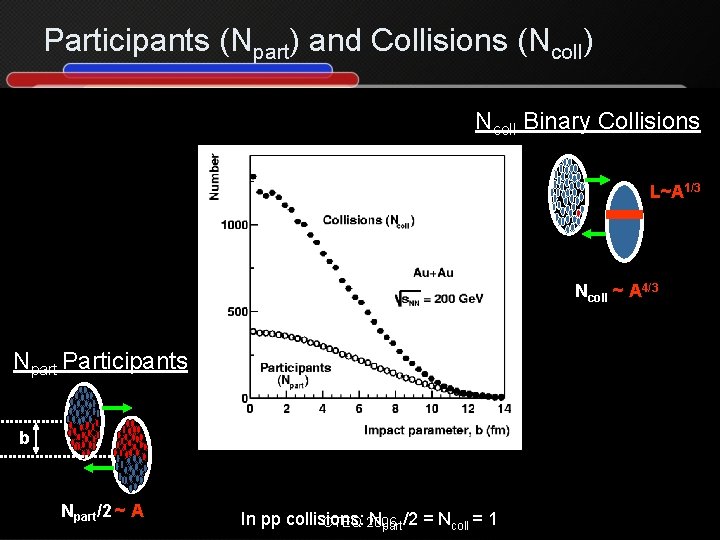
Participants (Npart) and Collisions (Ncoll) Ncoll Binary Collisions L~A 1/3 Ncoll ~ A 4/3 Npart Participants b Npart/2 ~ A In pp collisions: Npart/2 = Ncoll = 1 CTEQ 2006
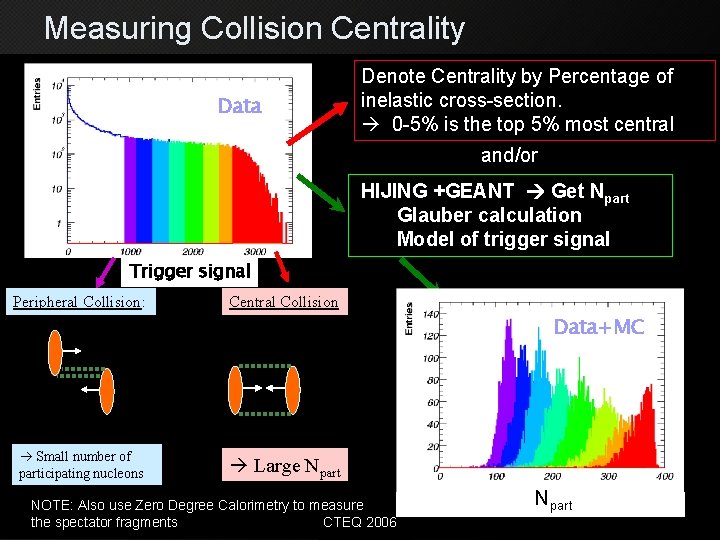
Measuring Collision Centrality Data Denote Centrality by Percentage of inelastic cross-section. 0 -5% is the top 5% most central and/or HIJING +GEANT Get Npart Glauber calculation Model of trigger signal Trigger signal Peripheral Collision: Central Collision Data+MC Small number of participating nucleons Large Npart NOTE: Also use Zero Degree Calorimetry to measure CTEQ 2006 the spectator fragments Npart
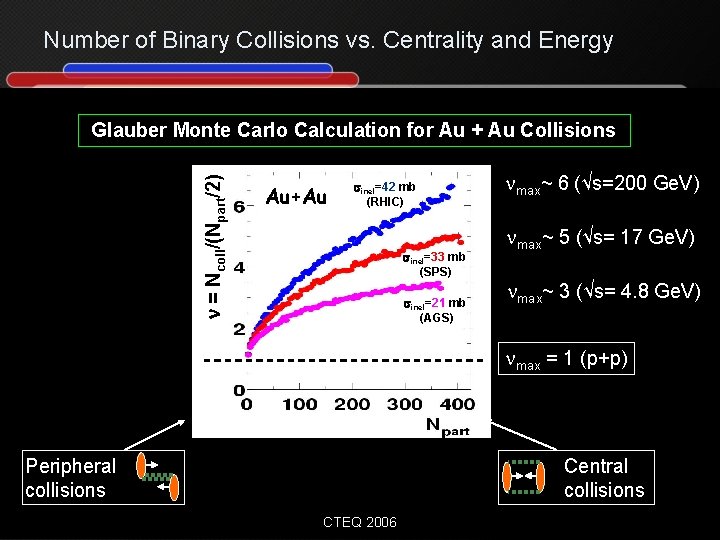
Number of Binary Collisions vs. Centrality and Energy n = Ncoll/(Npart/2) Glauber Monte Carlo Calculation for Au + Au Collisions Au+Au sinel=42 mb (RHIC) sinel=33 mb (SPS) sinel=21 mb (AGS) nmax~ 6 (√s=200 Ge. V) nmax~ 5 (√s= 17 Ge. V) nmax~ 3 (√s= 4. 8 Ge. V) nmax = 1 (p+p) Peripheral collisions Central collisions CTEQ 2006
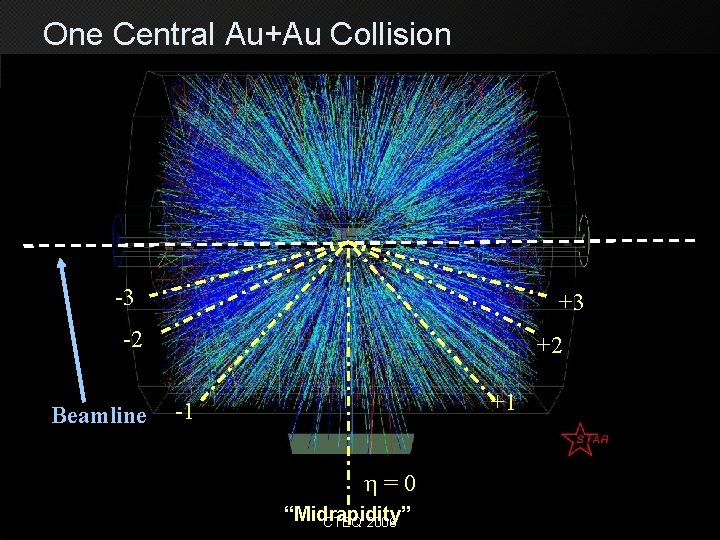
One Central Au+Au Collision -3 +3 -2 Beamline +2 +1 -1 h=0 “Midrapidity” CTEQ 2006
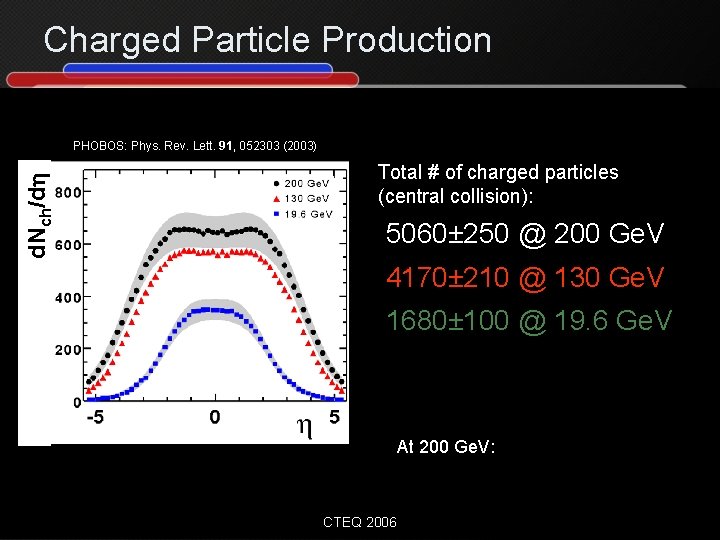
Charged Particle Production d. Nch/dh PHOBOS: Phys. Rev. Lett. 91, 052303 (2003) h=-ln tan q/2 Total # of charged particles (central collision): 5060± 250 @ 200 Ge. V 4170± 210 @ 130 Ge. V 1680± 100 @ 19. 6 Ge. V At 200 Ge. V: CTEQ 2006
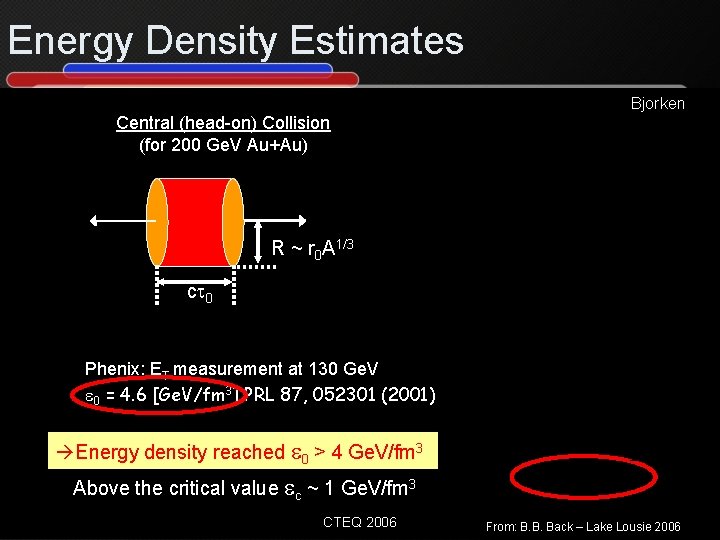
Energy Density Estimates Bjorken Central (head-on) Collision (for 200 Ge. V Au+Au) R ~ r 0 A 1/3 ct 0 Phenix: ET measurement at 130 Ge. V e 0 = 4. 6 [Ge. V/fm 3] PRL 87, 052301 (2001) Energy density reached e 0 > 4 Ge. V/fm 3 Above the critical value ec ~ 1 Ge. V/fm 3 CTEQ 2006 From: B. B. Back – Lake Lousie 2006
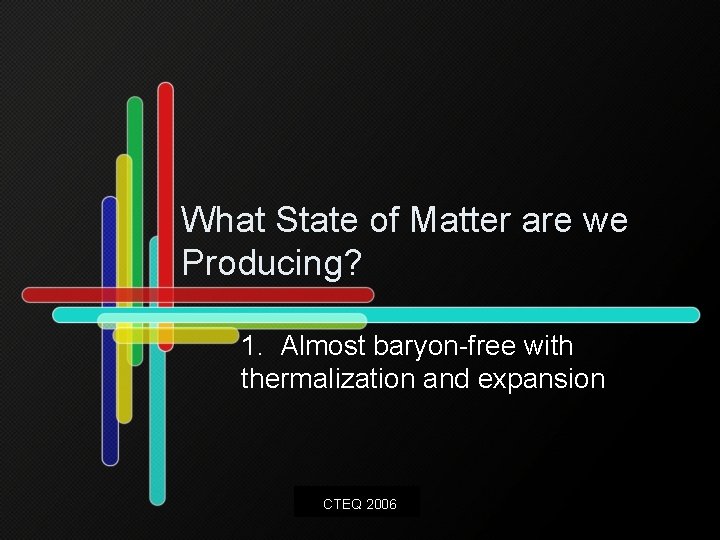
What State of Matter are we Producing? 1. Almost baryon-free with thermalization and expansion CTEQ 2006
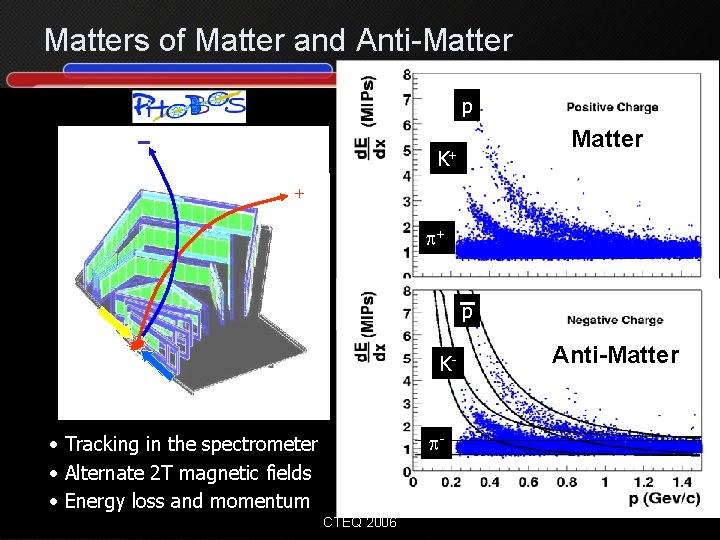
Matters of Matter and Anti-Matter p Matter K+ + p+ p K- p- • Tracking in the spectrometer • Alternate 2 T magnetic fields • Energy loss and momentum CTEQ 2006 Anti-Matter
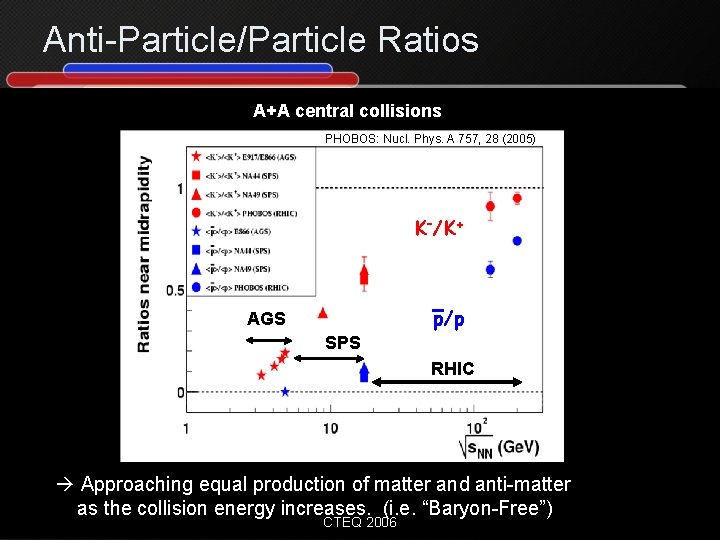
Anti-Particle/Particle Ratios A+A central collisions PHOBOS: Nucl. Phys. A 757, 28 (2005) K–/K+ p/p AGS SPS RHIC Approaching equal production of matter and anti-matter as the collision energy increases. (i. e. “Baryon-Free”) CTEQ 2006
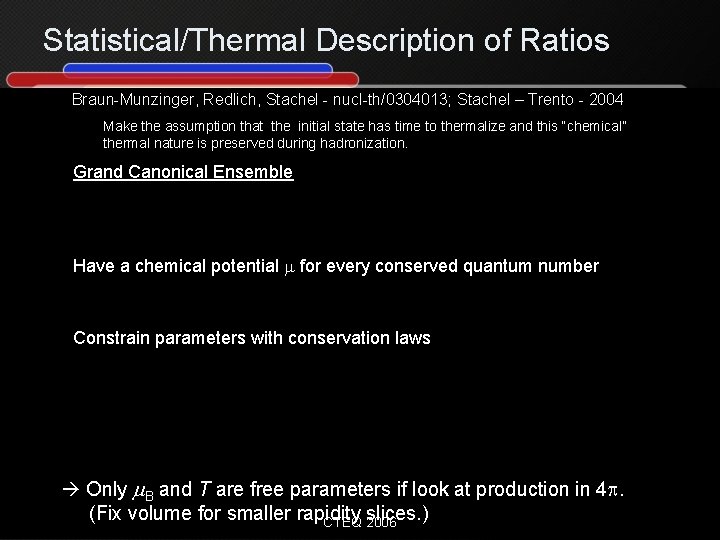
Statistical/Thermal Description of Ratios Braun-Munzinger, Redlich, Stachel - nucl-th/0304013; Stachel – Trento - 2004 Make the assumption that the initial state has time to thermalize and this “chemical” thermal nature is preserved during hadronization. Grand Canonical Ensemble Have a chemical potential m for every conserved quantum number Constrain parameters with conservation laws Only m. B and T are free parameters if look at production in 4 p. (Fix volume for smaller rapidity slices. ) CTEQ 2006
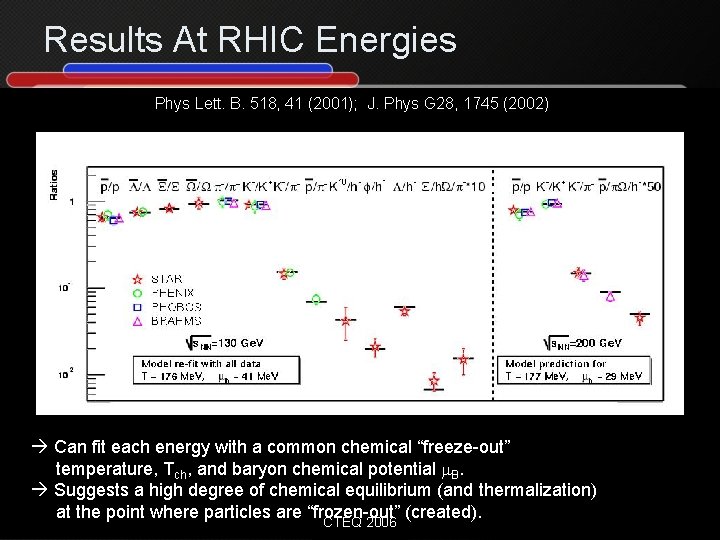
Results At RHIC Energies Phys Lett. B. 518, 41 (2001); J. Phys G 28, 1745 (2002) Can fit each energy with a common chemical “freeze-out” temperature, Tch, and baryon chemical potential m. B. Suggests a high degree of chemical equilibrium (and thermalization) at the point where particles are “frozen-out” (created). CTEQ 2006
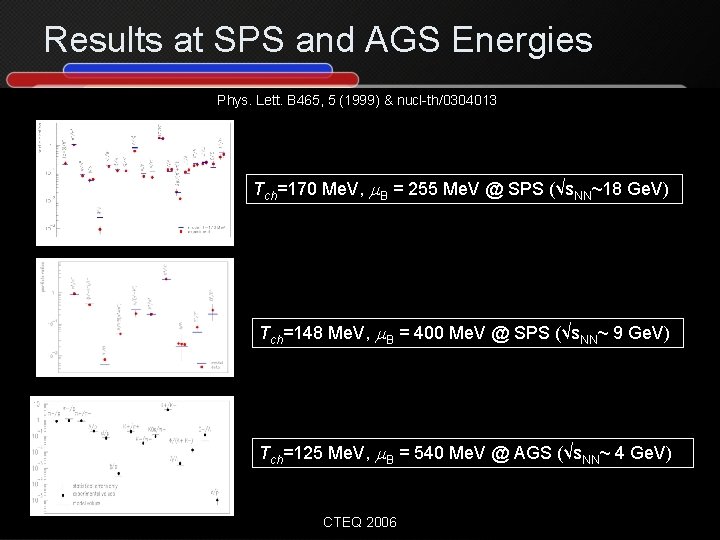
Results at SPS and AGS Energies Phys. Lett. B 465, 5 (1999) & nucl-th/0304013 Tch=170 Me. V, m. B = 255 Me. V @ SPS (√s. NN~18 Ge. V) Tch=148 Me. V, m. B = 400 Me. V @ SPS (√s. NN~ 9 Ge. V) Tch=125 Me. V, m. B = 540 Me. V @ AGS (√s. NN~ 4 Ge. V) CTEQ 2006
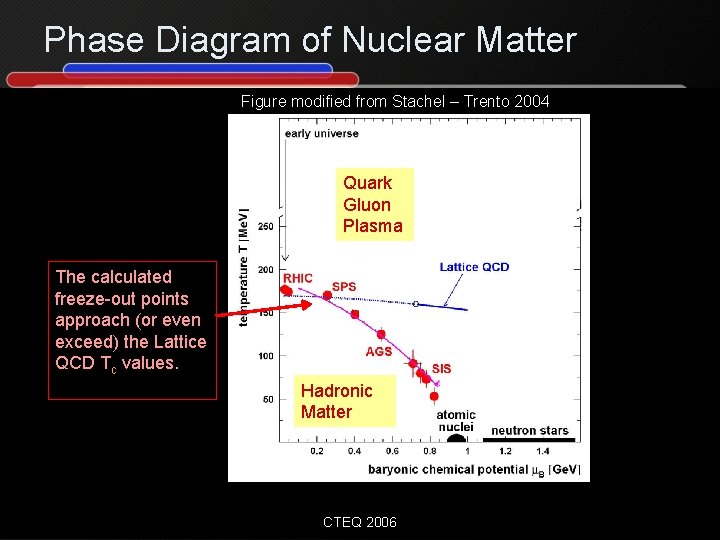
Phase Diagram of Nuclear Matter Figure modified from Stachel – Trento 2004 Quark Gluon Plasma The calculated freeze-out points approach (or even exceed) the Lattice QCD Tc values. Hadronic Matter CTEQ 2006
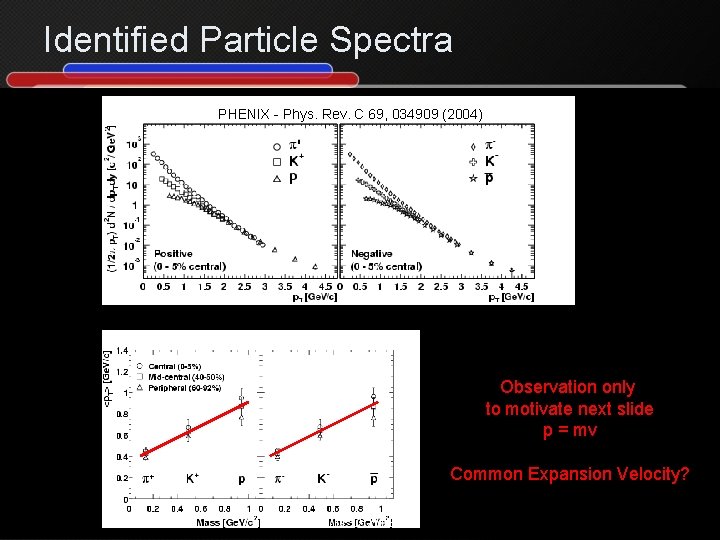
Identified Particle Spectra PHENIX - Phys. Rev. C 69, 034909 (2004) Observation only to motivate next slide p = mv Common Expansion Velocity? CTEQ 2006
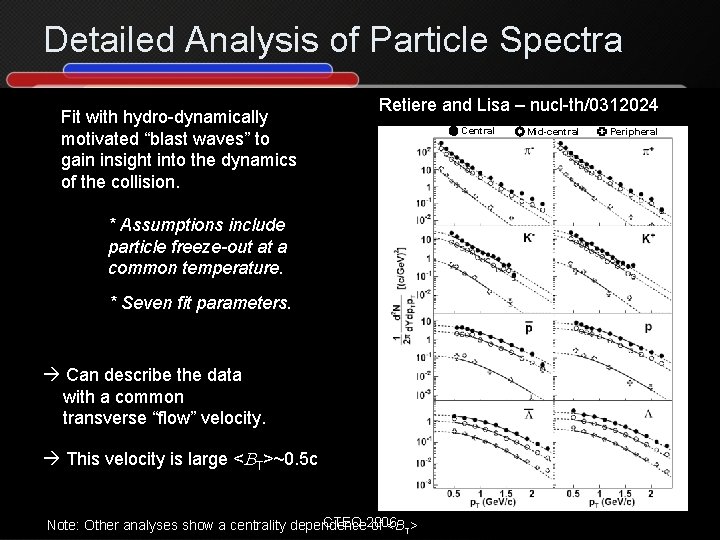
Detailed Analysis of Particle Spectra Fit with hydro-dynamically motivated “blast waves” to gain insight into the dynamics of the collision. Retiere and Lisa – nucl-th/0312024 * Assumptions include particle freeze-out at a common temperature. * Seven fit parameters. Can describe the data with a common transverse “flow” velocity. This velocity is large <BT>~0. 5 c CTEQ 2006 Note: Other analyses show a centrality dependence of <BT> Central Mid-central Peripheral
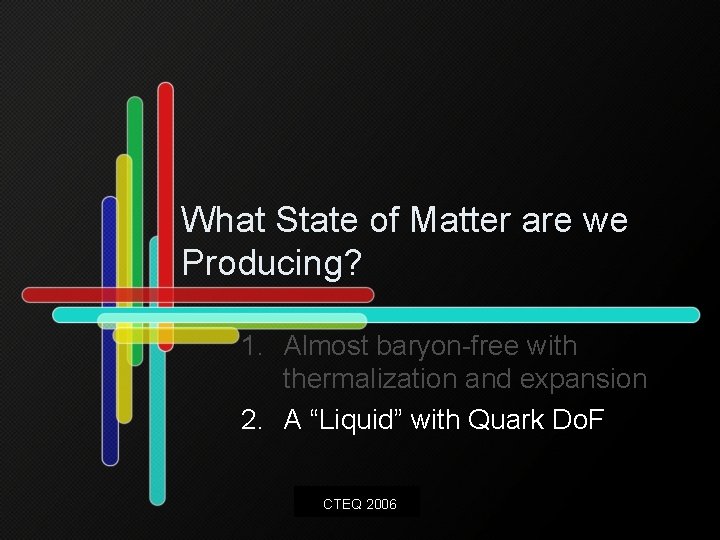
What State of Matter are we Producing? 1. Almost baryon-free with thermalization and expansion 2. A “Liquid” with Quark Do. F CTEQ 2006
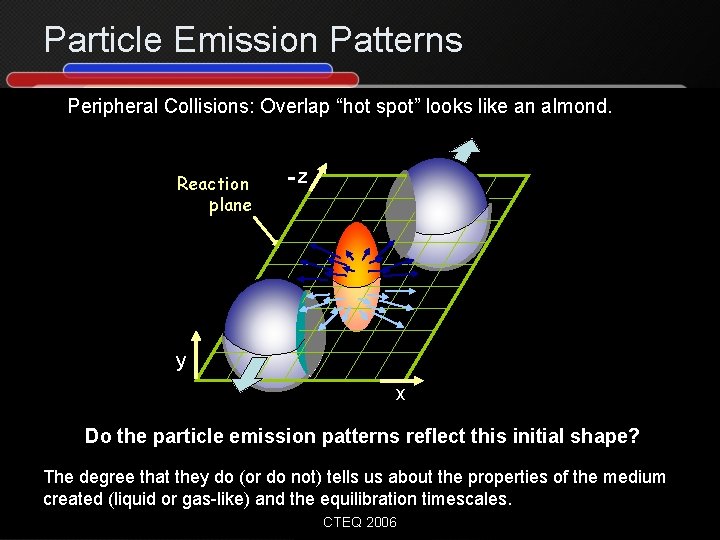
Particle Emission Patterns Peripheral Collisions: Overlap “hot spot” looks like an almond. Reaction plane z y x Do the particle emission patterns reflect this initial shape? The degree that they do (or do not) tells us about the properties of the medium created (liquid or gas-like) and the equilibration timescales. CTEQ 2006
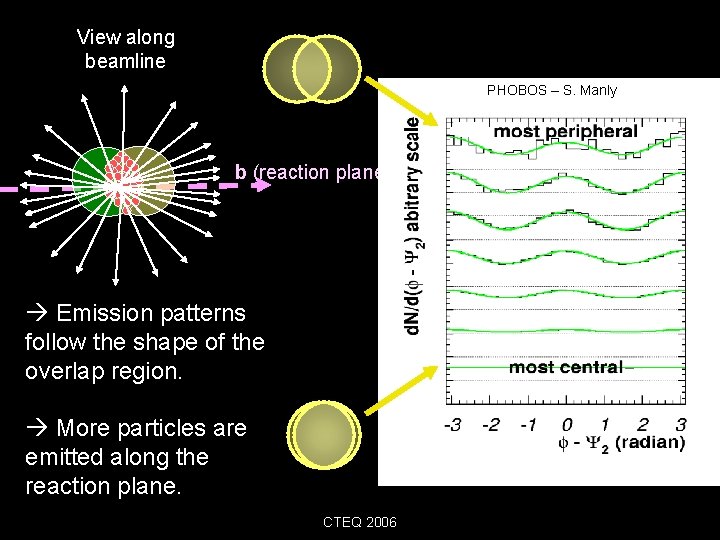
View along beamline PHOBOS – S. Manly b (reaction plane) Emission patterns follow the shape of the overlap region. More particles are emitted along the reaction plane. CTEQ 2006
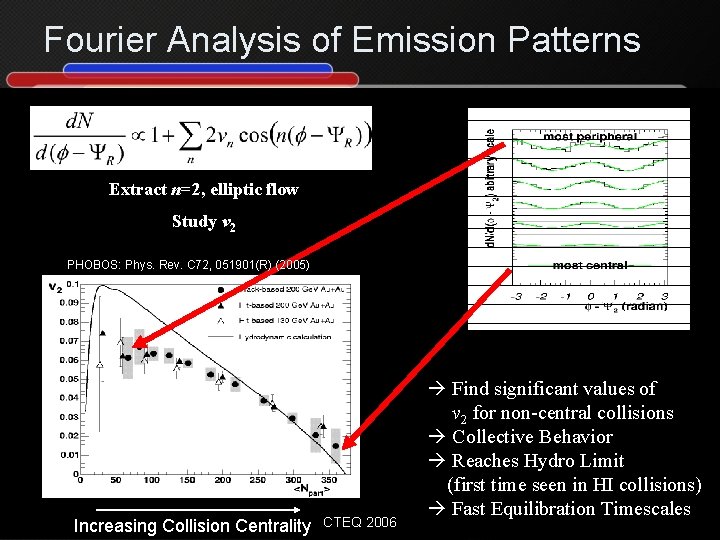
Fourier Analysis of Emission Patterns Extract n=2, elliptic flow Study v 2 PHOBOS: Phys. Rev. C 72, 051901(R) (2005) Increasing Collision Centrality CTEQ 2006 Find significant values of v 2 for non-central collisions Collective Behavior Reaches Hydro Limit (first time seen in HI collisions) Fast Equilibration Timescales
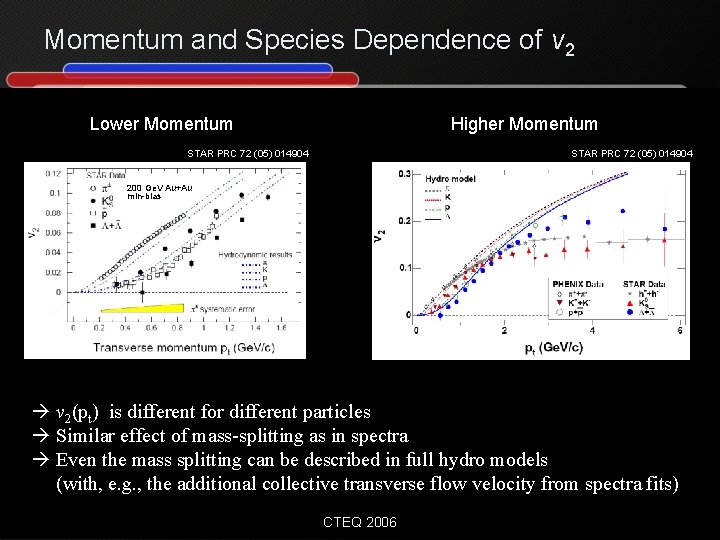
Momentum and Species Dependence of v 2 Lower Momentum Higher Momentum STAR PRC 72 (05) 014904 200 Ge. V Au+Au min-bias v 2(pt) is different for different particles Similar effect of mass-splitting as in spectra Even the mass splitting can be described in full hydro models (with, e. g. , the additional collective transverse flow velocity from spectra fits) CTEQ 2006
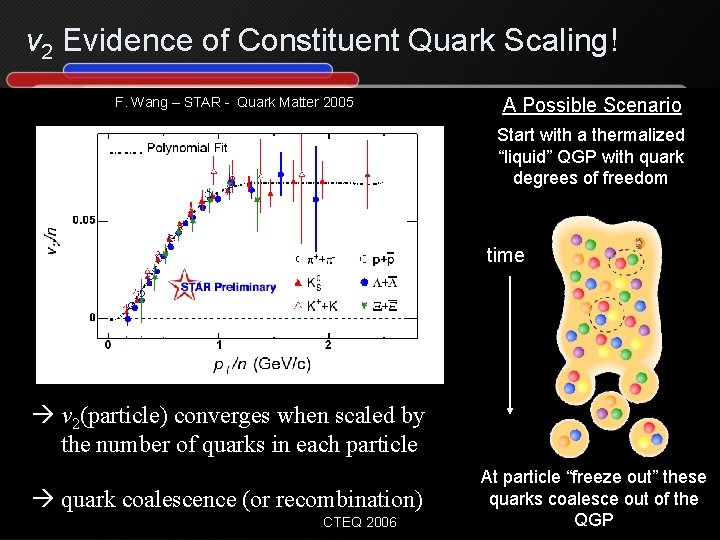
v 2 Evidence of Constituent Quark Scaling! F. Wang – STAR - Quark Matter 2005 A Possible Scenario Start with a thermalized “liquid” QGP with quark degrees of freedom time v 2(particle) converges when scaled by the number of quarks in each particle quark coalescence (or recombination) CTEQ 2006 At particle “freeze out” these quarks coalesce out of the QGP
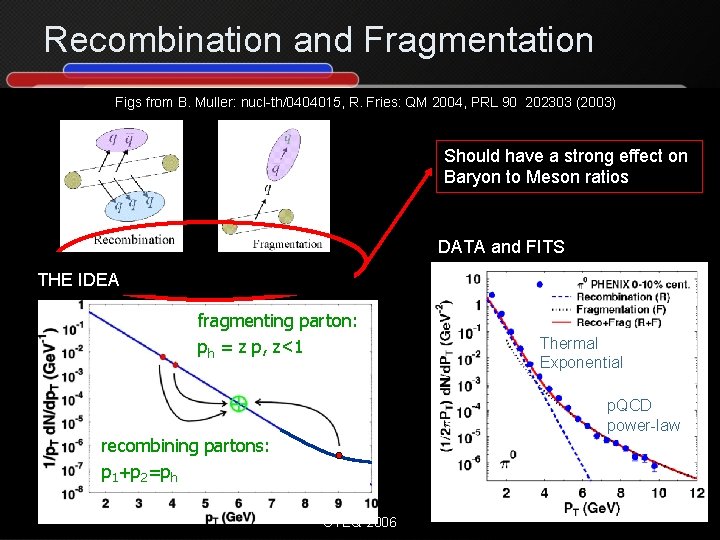
Recombination and Fragmentation Figs from B. Muller: nucl-th/0404015, R. Fries: QM 2004, PRL 90 202303 (2003) Should have a strong effect on Baryon to Meson ratios DATA and FITS THE IDEA fragmenting parton: ph = z p, z<1 Thermal Exponential p. QCD power-law recombining partons: p 1+p 2=ph CTEQ 2006
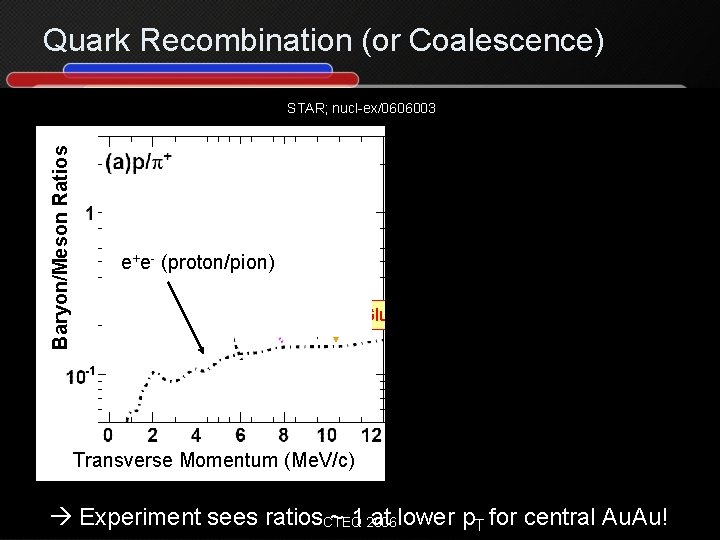
Quark Recombination (or Coalescence) Baryon/Meson Ratios STAR; nucl-ex/0606003 e+e- (proton/pion) Gluon Jets? Transverse Momentum (Me. V/c) Experiment sees ratios. CTEQ ~ 1 2006 at lower p. T for central Au. Au!
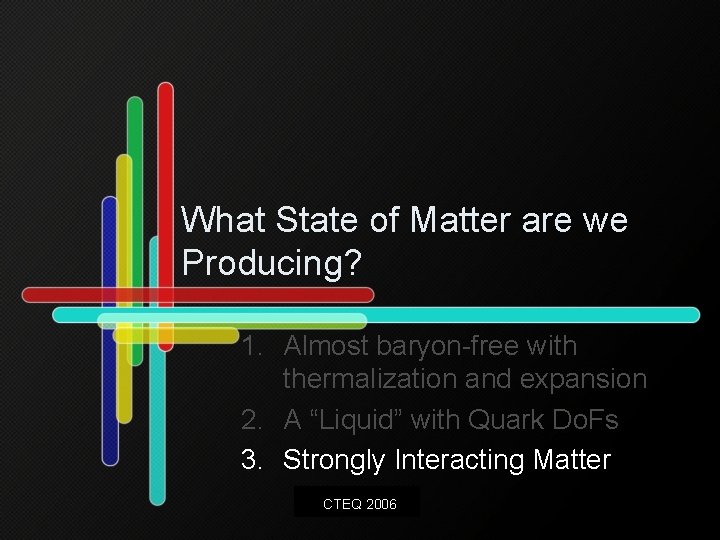
What State of Matter are we Producing? 1. Almost baryon-free with thermalization and expansion 2. A “Liquid” with Quark Do. Fs 3. Strongly Interacting Matter CTEQ 2006
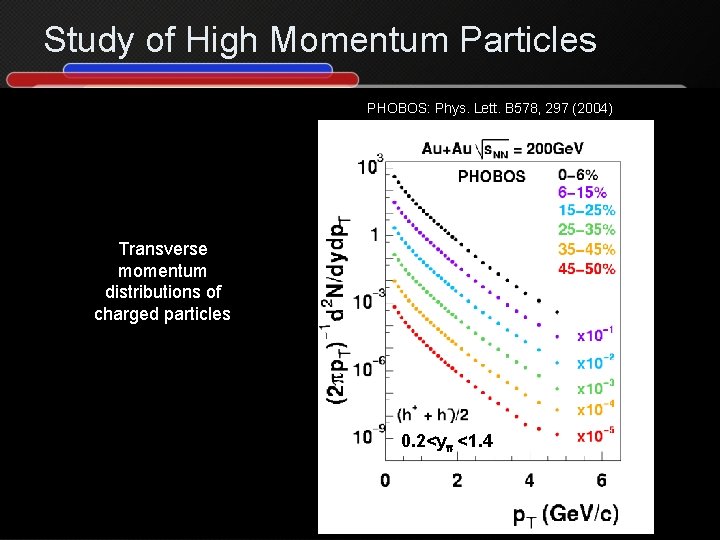
Study of High Momentum Particles PHOBOS: Phys. Lett. B 578, 297 (2004) Transverse momentum distributions of charged particles 0. 2<yp <1. 4 CTEQ 2006
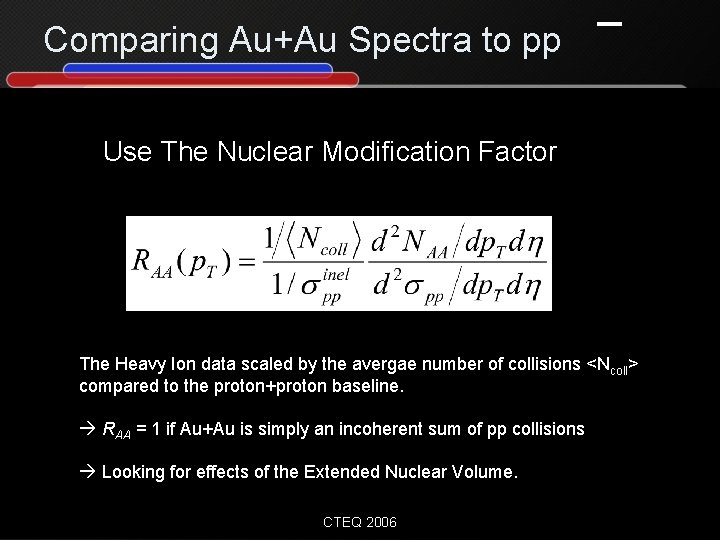
Comparing Au+Au Spectra to pp Use The Nuclear Modification Factor The Heavy Ion data scaled by the avergae number of collisions <Ncoll> compared to the proton+proton baseline. RAA = 1 if Au+Au is simply an incoherent sum of pp collisions Looking for effects of the Extended Nuclear Volume. CTEQ 2006
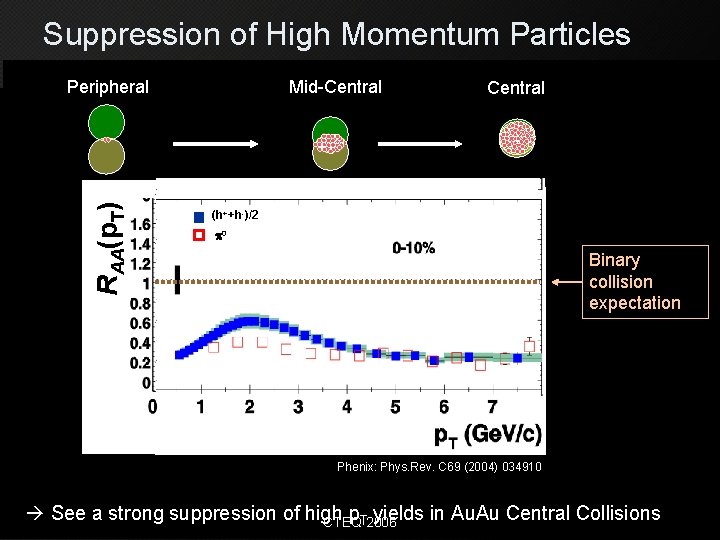
Suppression of High Momentum Particles RAA(p. T) Peripheral Mid-Central (h++h-)/2 p 0 Binary collision expectation Phenix: Phys. Rev. C 69 (2004) 034910 See a strong suppression of high p yields in Au. Au Central Collisions CTEQT 2006
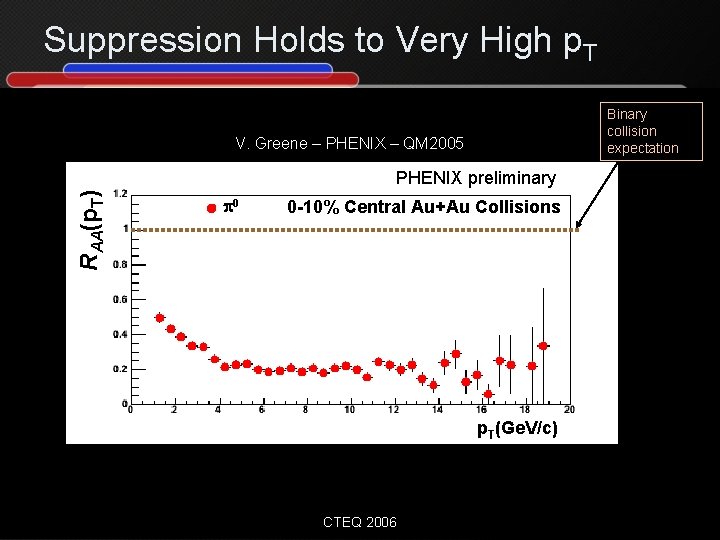
Suppression Holds to Very High p. T Binary collision expectation V. Greene – PHENIX – QM 2005 RAA(p. T) PHENIX preliminary p 0 0 -10% Central Au+Au Collisions p. T(Ge. V/c) CTEQ 2006
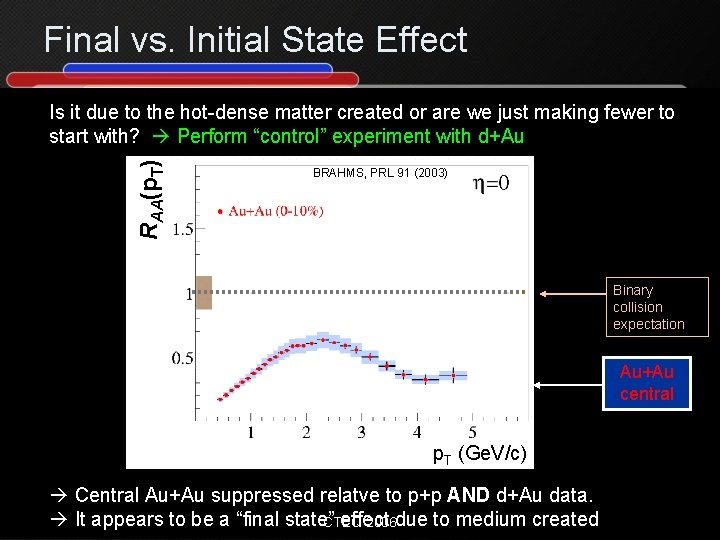
Final vs. Initial State Effect RAA(p. T) Is it due to the hot-dense matter created or are we just making fewer to start with? Perform “control” experiment with d+Au BRAHMS, PRL 91 (2003) d+Au Binary collision expectation Au+Au central p. T (Ge. V/c) Central Au+Au suppressed relatve to p+p AND d+Au data. It appears to be a “final state” effect CTEQ 2006 due to medium created
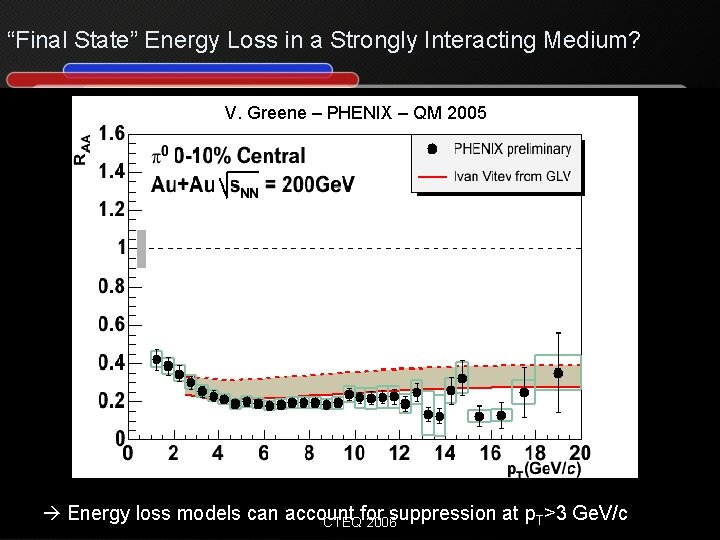
“Final State” Energy Loss in a Strongly Interacting Medium? V. Greene – PHENIX – QM 2005 Energy loss models can account for suppression at p. T>3 Ge. V/c CTEQ 2006
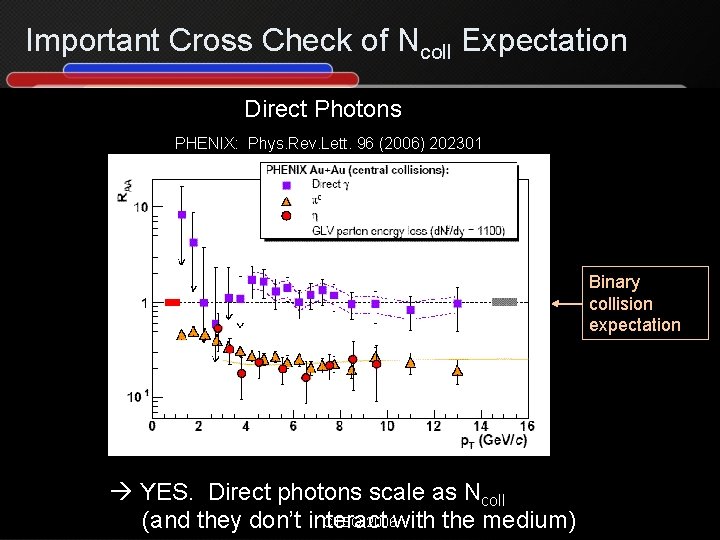
Important Cross Check of Ncoll Expectation Direct Photons PHENIX: Phys. Rev. Lett. 96 (2006) 202301 Binary collision expectation YES. Direct photons scale as Ncoll CTEQ 2006 with the medium) (and they don’t interact
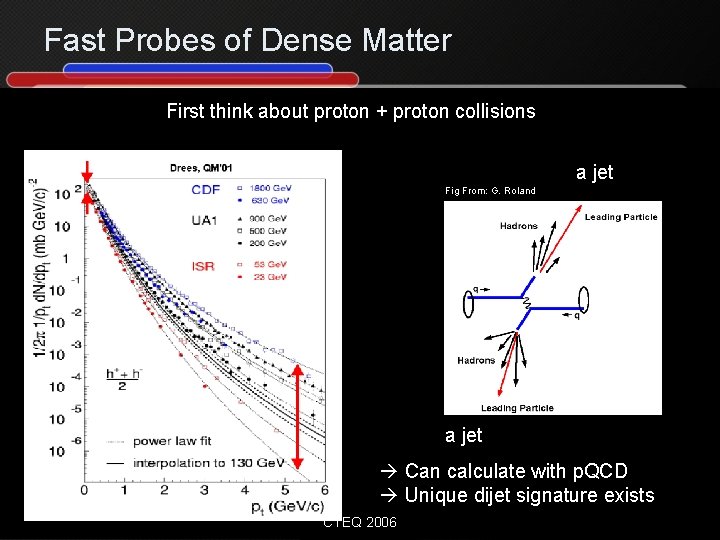
Fast Probes of Dense Matter First think about proton + proton collisions a jet Fig From: G. Roland a jet Can calculate with p. QCD Unique dijet signature exists CTEQ 2006
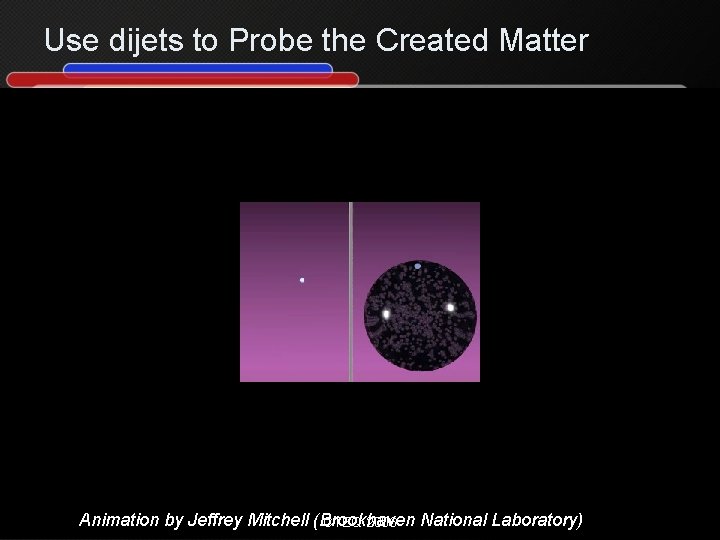
Use dijets to Probe the Created Matter Animation by Jeffrey Mitchell (Brookhaven National Laboratory) CTEQ 2006
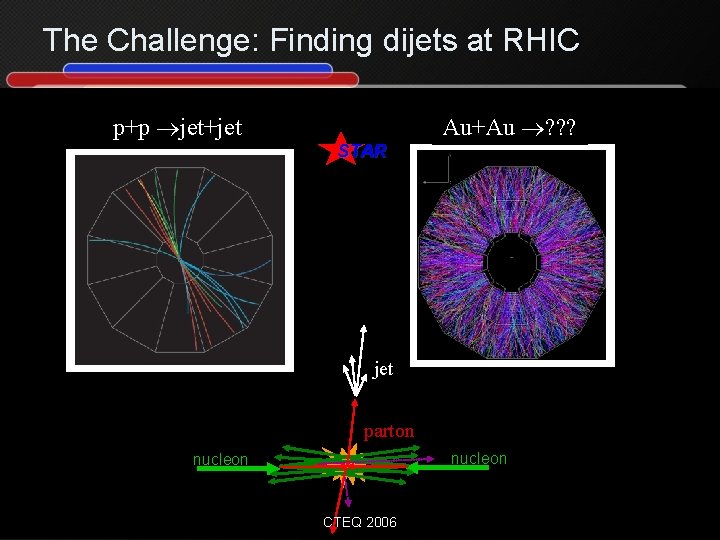
The Challenge: Finding dijets at RHIC p+p jet+jet Au+Au ? ? ? STAR jet parton nucleon CTEQ 2006
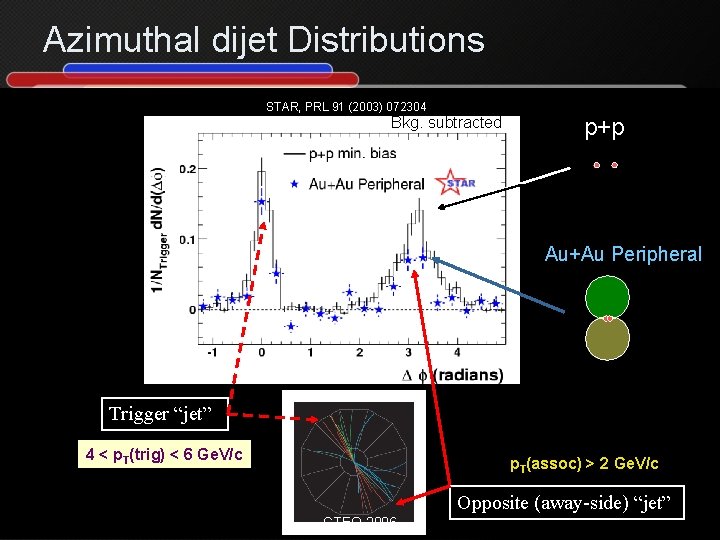
Azimuthal dijet Distributions STAR, PRL 91 (2003) 072304 Bkg. subtracted p+p Au+Au Peripheral Trigger “jet” 4 < p. T(trig) < 6 Ge. V/c p. T(assoc) > 2 Ge. V/c Opposite (away-side) “jet” CTEQ 2006
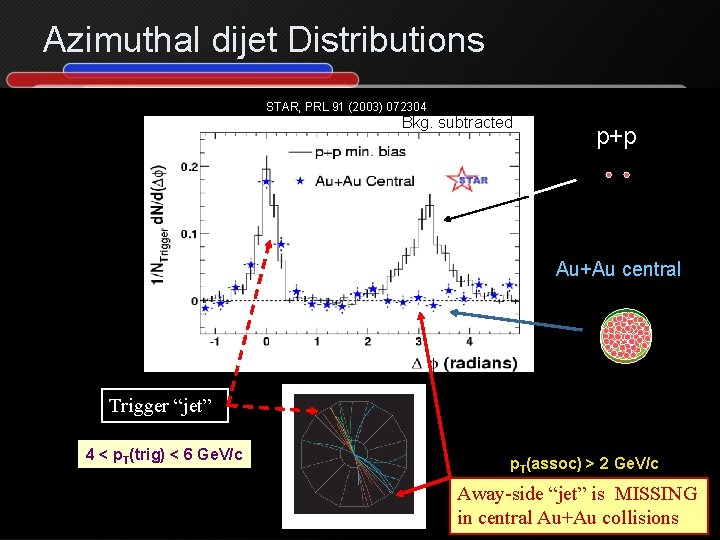
Azimuthal dijet Distributions STAR, PRL 91 (2003) 072304 Bkg. subtracted p+p Au+Au central Trigger “jet” 4 < p. T(trig) < 6 Ge. V/c p. T(assoc) > 2 Ge. V/c CTEQ 2006 Away-side “jet” is MISSING in central Au+Au collisions
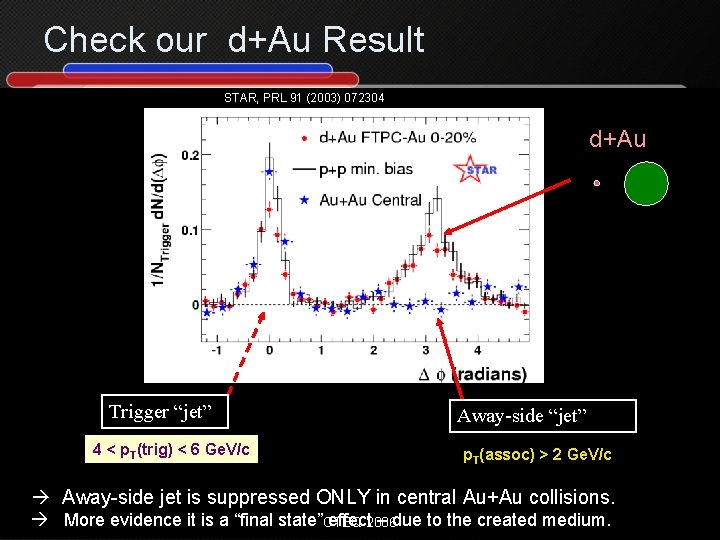
Check our d+Au Result STAR, PRL 91 (2003) 072304 d+Au Trigger “jet” 4 < p. T(trig) < 6 Ge. V/c Away-side “jet” p. T(assoc) > 2 Ge. V/c Away-side jet is suppressed ONLY in central Au+Au collisions. More evidence it is a “final state”CTEQ effect 2006 – due to the created medium.
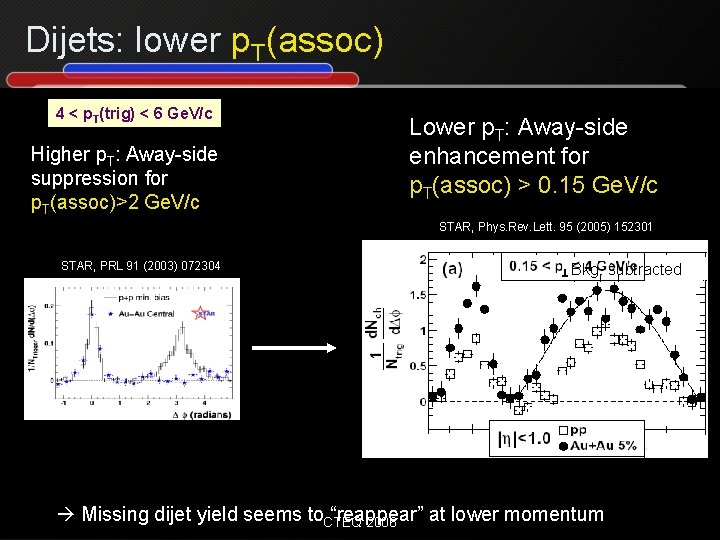
Dijets: lower p. T(assoc) 4 < p. T(trig) < 6 Ge. V/c Higher p. T: Away-side suppression for p. T(assoc)>2 Ge. V/c Lower p. T: Away-side enhancement for p. T(assoc) > 0. 15 Ge. V/c STAR, Phys. Rev. Lett. 95 (2005) 152301 STAR, PRL 91 (2003) 072304 Bkg. subtracted Missing dijet yield seems to. CTEQ “reappear” at lower momentum 2006
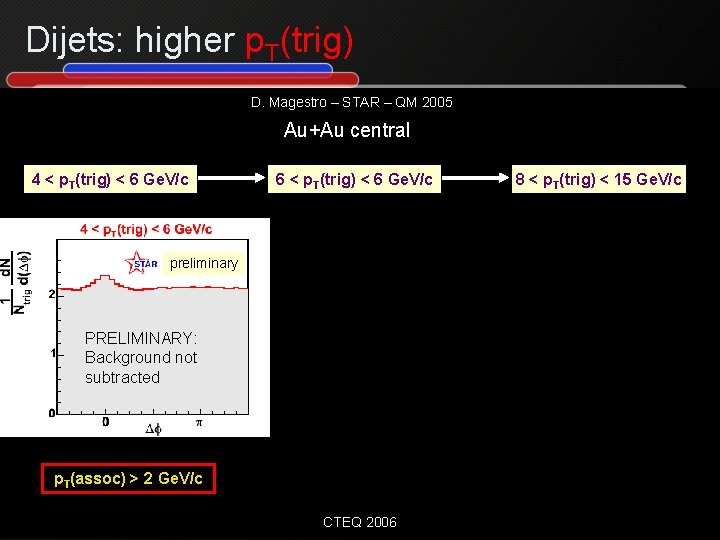
Dijets: higher p. T(trig) D. Magestro – STAR – QM 2005 Au+Au central 4 < p. T(trig) < 6 Ge. V/c 6 < p. T(trig) < 6 Ge. V/c preliminary PRELIMINARY: Background not subtracted p. T(assoc) > 2 Ge. V/c CTEQ 2006 8 < p. T(trig) < 15 Ge. V/c
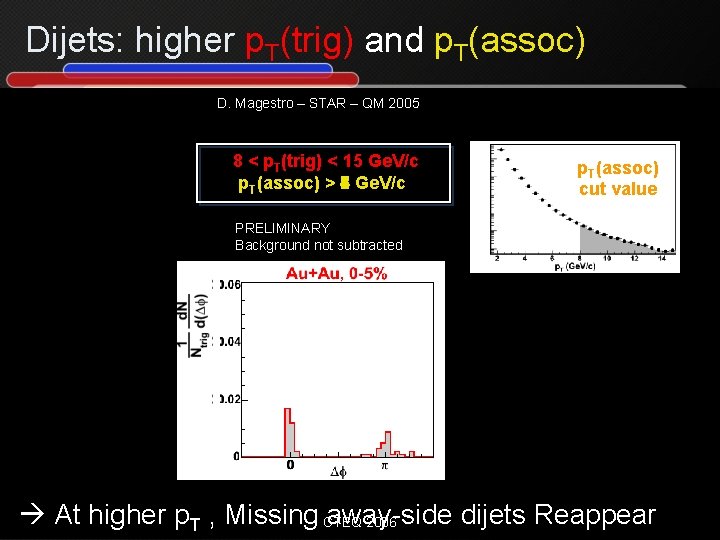
Dijets: higher p. T(trig) and p. T(assoc) D. Magestro – STAR – QM 2005 8 < p. T(trig) < 15 Ge. V/c p. T(assoc) > 8 2 Ge. V/c 3 4 5 6 7 p. T(assoc) cut value PRELIMINARY Background not subtracted At higher p. T , Missing CTEQ away-side dijets Reappear 2006
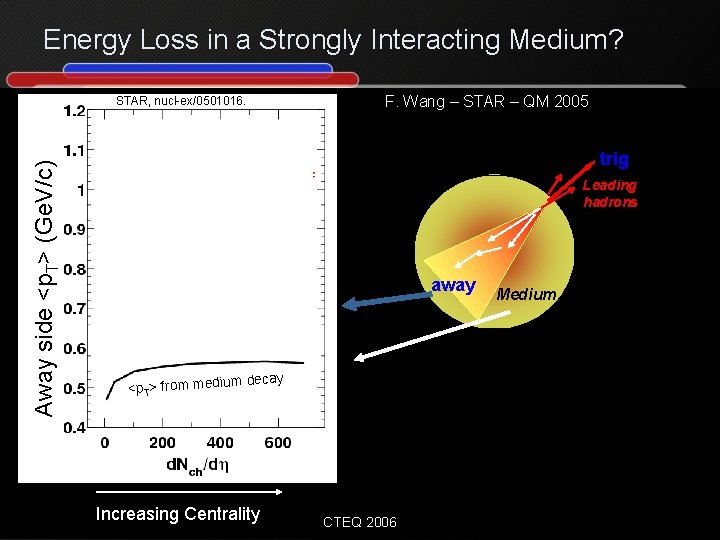
Energy Loss in a Strongly Interacting Medium? F. Wang – STAR – QM 2005 Away side <p. T> (Ge. V/c) STAR, nucl-ex/0501016. trig <p T> fro Leading hadrons ma wa y je ts away ecay md <p. T> from mediu Increasing Centrality CTEQ 2006 Medium
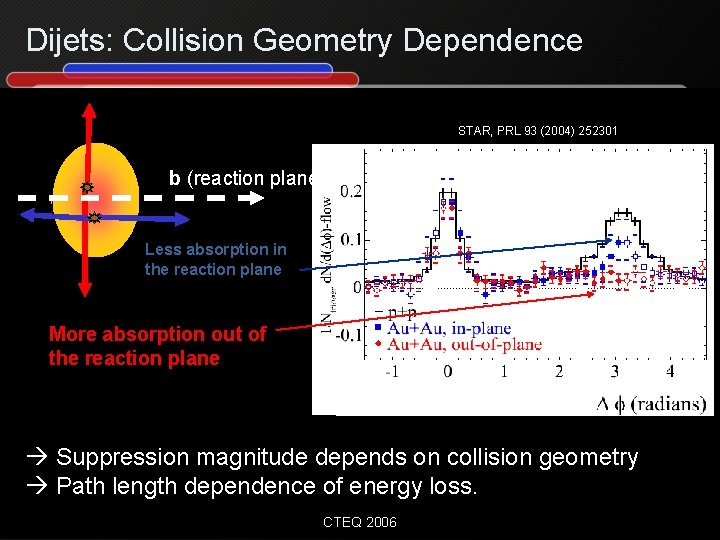
Dijets: Collision Geometry Dependence STAR, PRL 93 (2004) 252301 b (reaction plane) Less absorption in the reaction plane More absorption out of the reaction plane Suppression magnitude depends on collision geometry Path length dependence of energy loss. CTEQ 2006
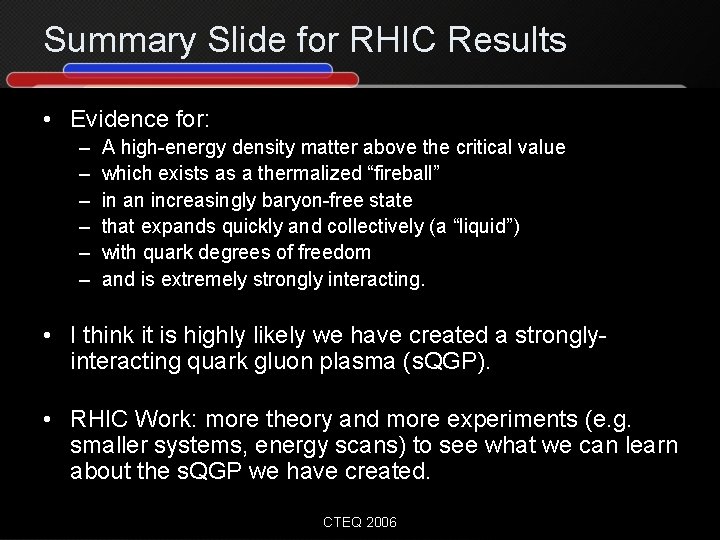
Summary Slide for RHIC Results • Evidence for: – – – A high-energy density matter above the critical value which exists as a thermalized “fireball” in an increasingly baryon-free state that expands quickly and collectively (a “liquid”) with quark degrees of freedom and is extremely strongly interacting. • I think it is highly likely we have created a stronglyinteracting quark gluon plasma (s. QGP). • RHIC Work: more theory and more experiments (e. g. smaller systems, energy scans) to see what we can learn about the s. QGP we have created. CTEQ 2006
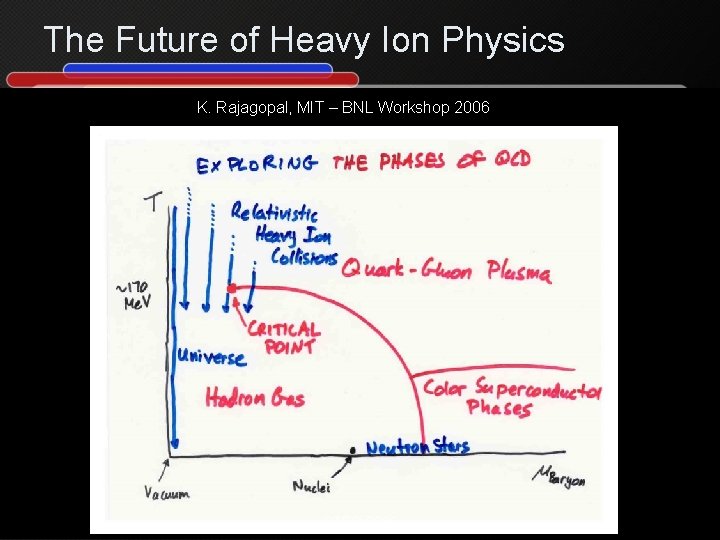
The Future of Heavy Ion Physics K. Rajagopal, MIT – BNL Workshop 2006 CTEQ 2006
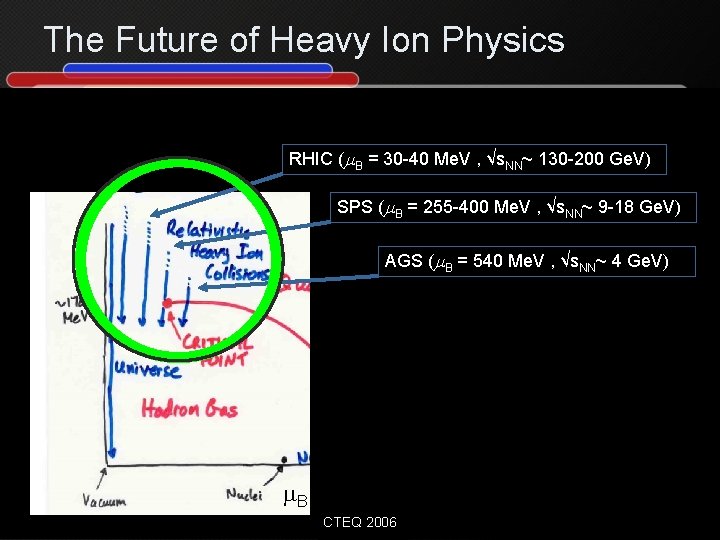
The Future of Heavy Ion Physics RHIC (m. B = 30 -40 Me. V , √s. NN~ 130 -200 Ge. V) SPS (m. B = 255 -400 Me. V , √s. NN~ 9 -18 Ge. V) AGS (m. B = 540 Me. V , √s. NN~ 4 Ge. V) m. B CTEQ 2006
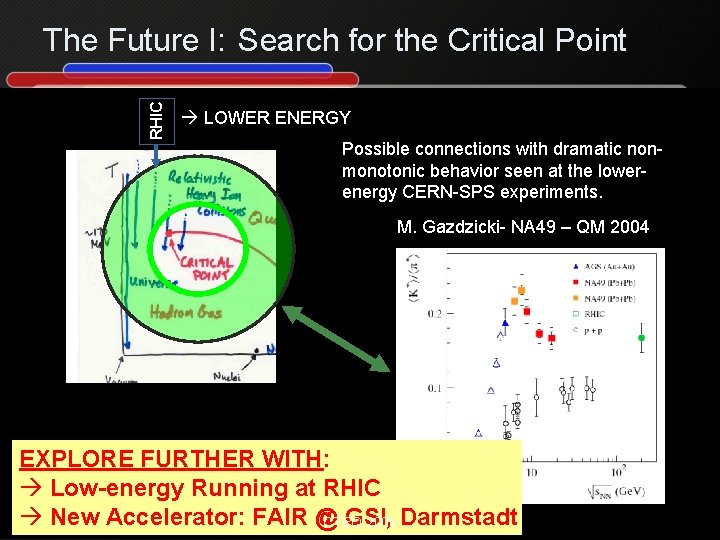
RHIC The Future I: Search for the Critical Point LOWER ENERGY Possible connections with dramatic nonmonotonic behavior seen at the lowerenergy CERN-SPS experiments. M. Gazdzicki- NA 49 – QM 2004 EXPLORE FURTHER WITH: Low-energy Running at RHIC New Accelerator: FAIR @ GSI, CTEQ 2006 Darmstadt
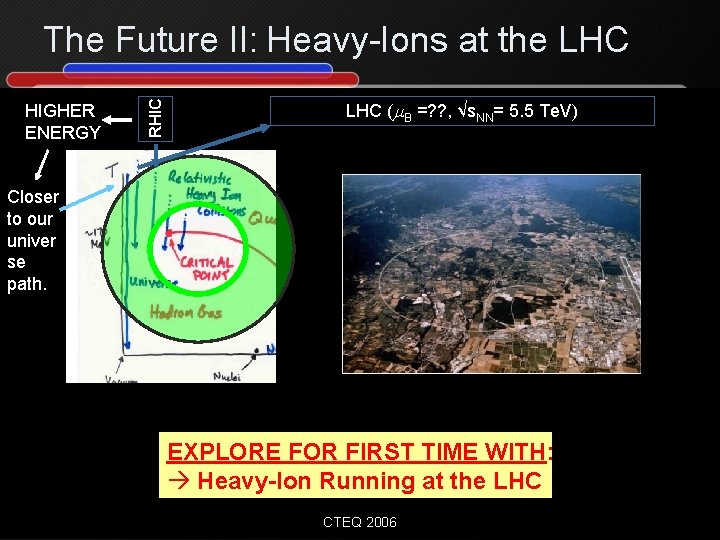
HIGHER ENERGY RHIC The Future II: Heavy-Ions at the LHC (m. B =? ? , √s. NN= 5. 5 Te. V) Closer to our univer se path. EXPLORE FOR FIRST TIME WITH: Heavy-Ion Running at the LHC CTEQ 2006
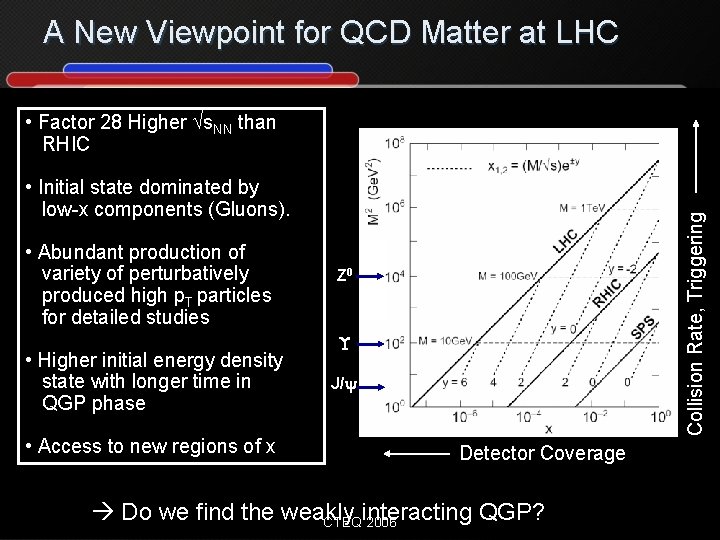
A New Viewpoint for QCD Matter at LHC • Factor 28 Higher s. NN than RHIC • Abundant production of variety of perturbatively produced high p. T particles for detailed studies • Higher initial energy density state with longer time in QGP phase • Access to new regions of x Collision Rate, Triggering • Initial state dominated by low-x components (Gluons). Z 0 J/ Detector Coverage Do we find the weakly QGP? CTEQinteracting 2006
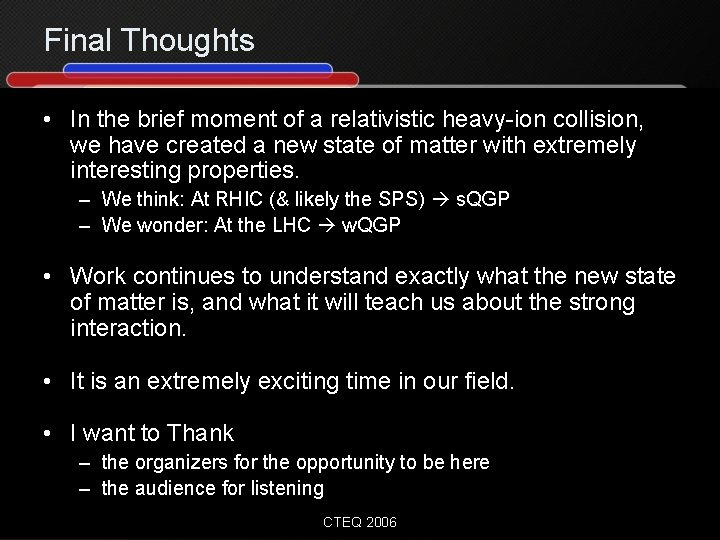
Final Thoughts • In the brief moment of a relativistic heavy-ion collision, we have created a new state of matter with extremely interesting properties. – We think: At RHIC (& likely the SPS) s. QGP – We wonder: At the LHC w. QGP • Work continues to understand exactly what the new state of matter is, and what it will teach us about the strong interaction. • It is an extremely exciting time in our field. • I want to Thank – the organizers for the opportunity to be here – the audience for listening CTEQ 2006
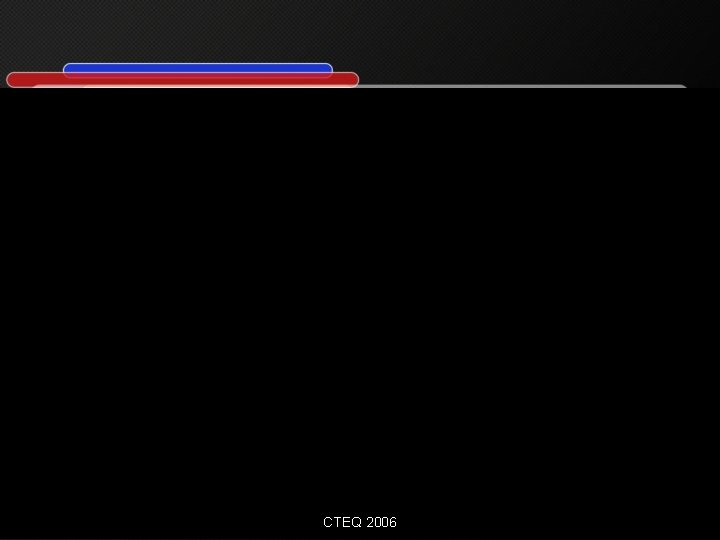
CTEQ 2006
David hofman uic
Impulse definition physics
Ratey method
Heavy ion
Nursing diagnosis for brain abscess
Harm hofman
Joe hofman
Uo brno
Stanislav hofman
Vijvertuinen ada hofman
Energia wiatrowa prezentacja
C6h12 fuerza intermolecular
Qumica
Fuerzas dipolo-dipolo ejemplos
Dispersion forces examples
Charm mesom
Types of collisions/ impulse graphs answer key
An estimated force time curve for a baseball
Types of collisions
In the defensive driver success formula, "i" refers too
How do we know momentum is conserved
A freight train is being assembled in a switching yard
Necessary for successful collisions to occur
Types of collisions
Inelastic collision examples real world
A moderate force will break an egg
Collisions and explosions
Collisions and explosions
Types of collision
Is momentum conserved in all collisions
As you enter the deceleration lane or the exit ramp
Drawbacks of free electron theory
What is collision frequency
To avoid collisions a defensive driver should
Prévention des collisions engins-piétons
Inelastic collision
Collisions
Chapter 6 momentum and collisions
üiç
Uic cs flowchart
Drc-101
üiç
Yağmur eraslan
Data extraction table example
Bing liu uic
Uic extranet
Uic healthcare administration
Uic cyber security
Ccts uic
Seo931
John lillis uic
Carlo naldi politecnico torino
Cs 583 uic
Bing liu uic
Kevin cisner
Uic exam schedule
Isabel cruz uic
Uic rrc
Extranet uic
Ece 465 uic
Ece 368
John lillis uic
Uic cme
Uic housing dock
Bing liu uic
Bing liu uic
Why does it happen
University physics with modern physics fifteenth edition
Ia physics examples
David skinner physics
Protun
What is density
Prolan heavy
Fiducary
Heavy equipment safety checklist