Electron cooling at the Recycler Update and Cooling
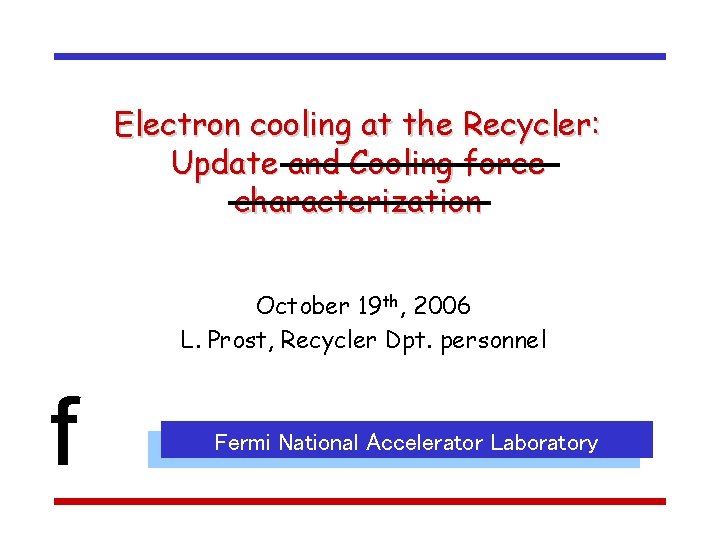
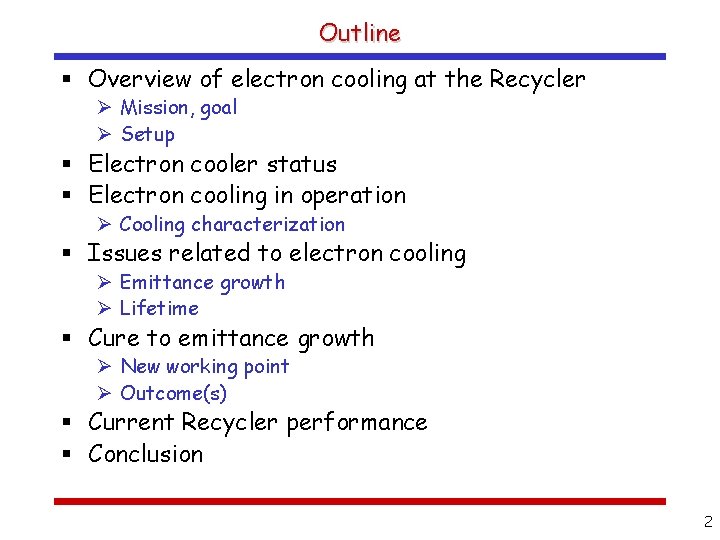
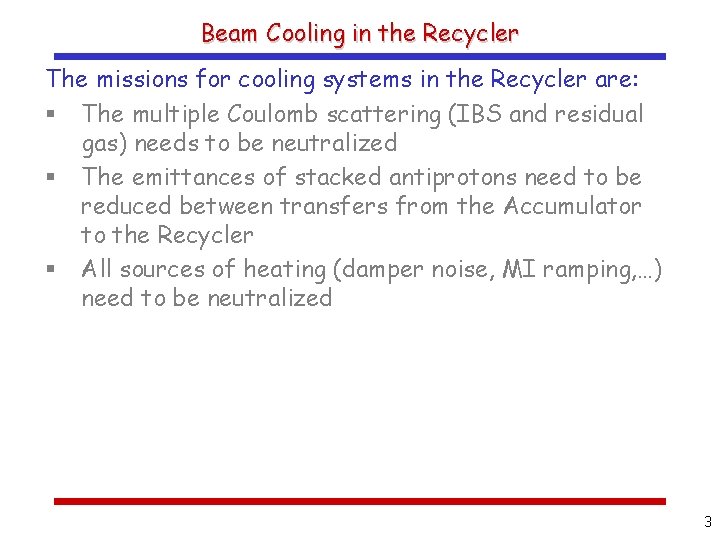
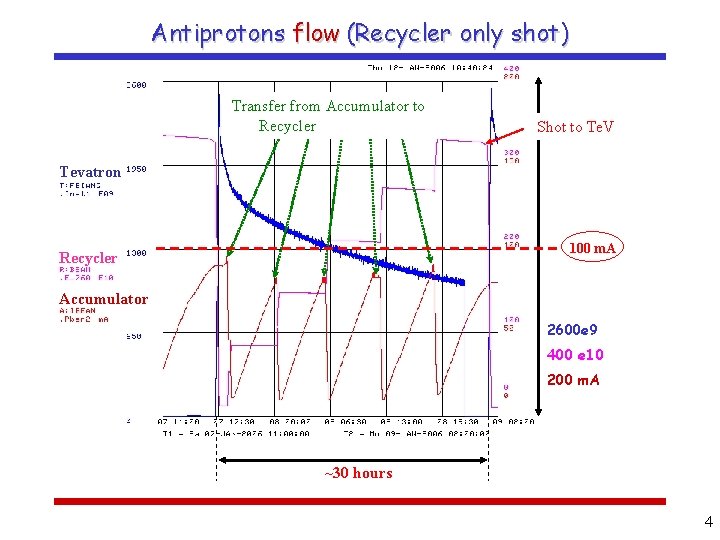
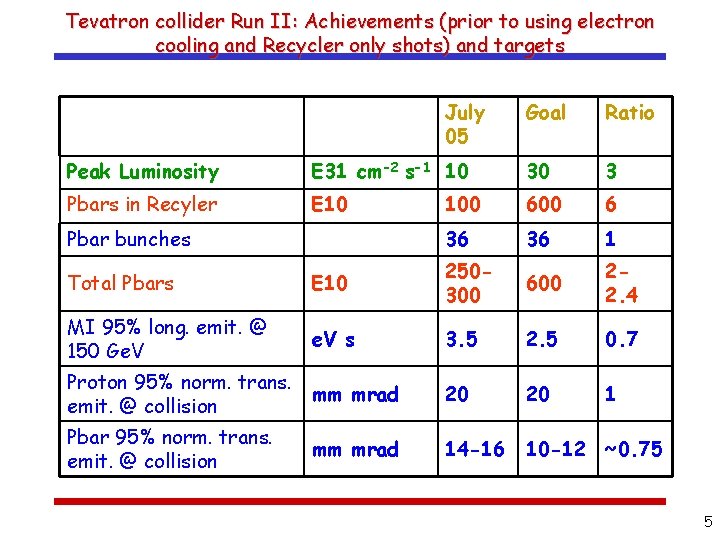
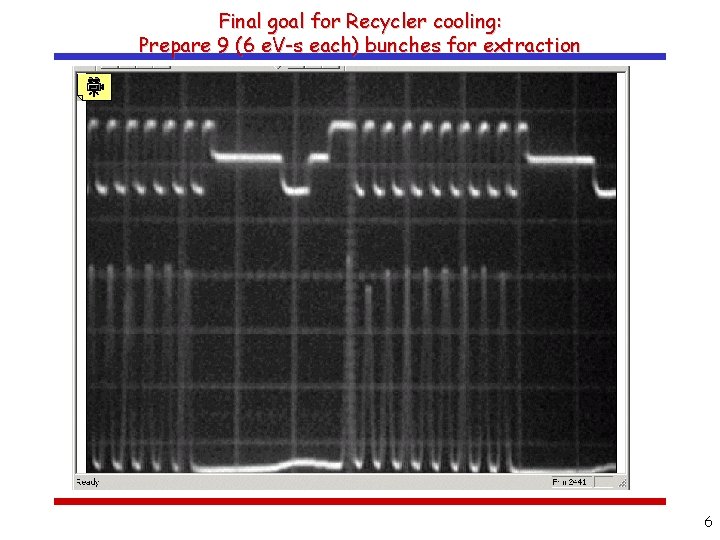
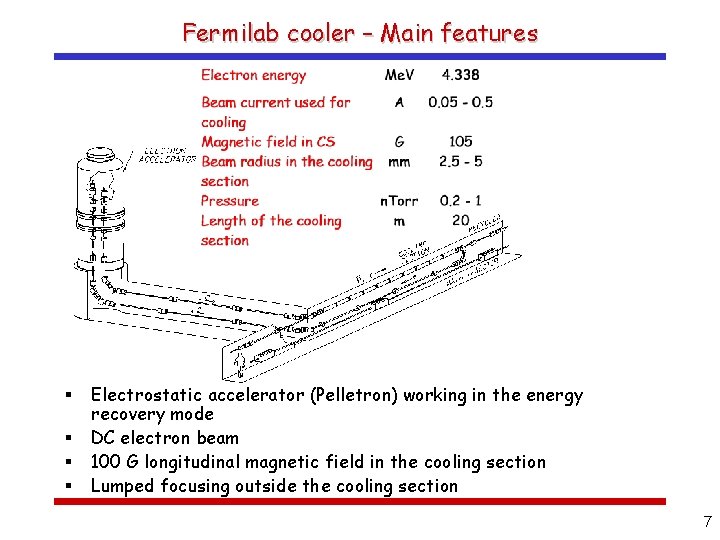
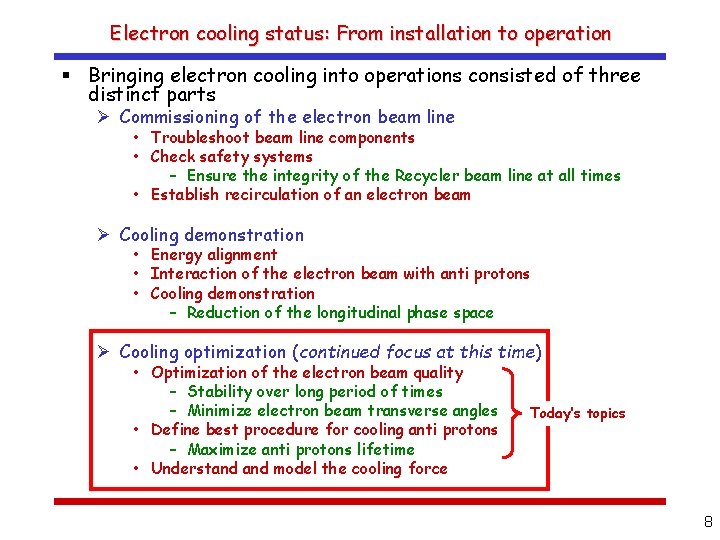
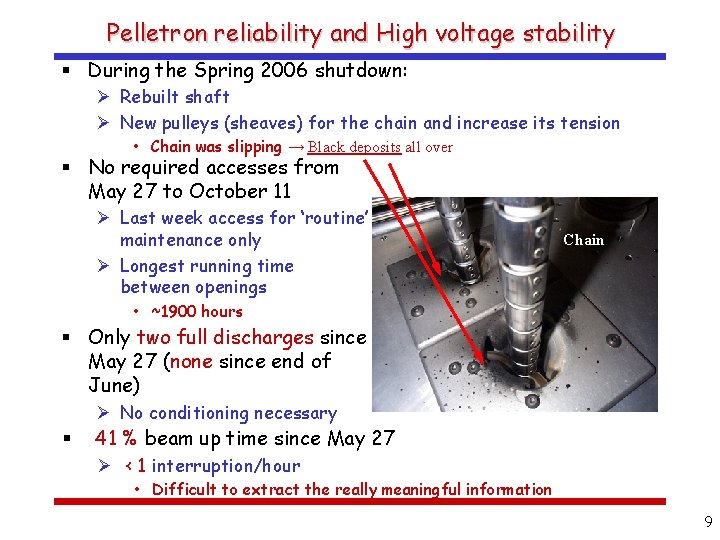
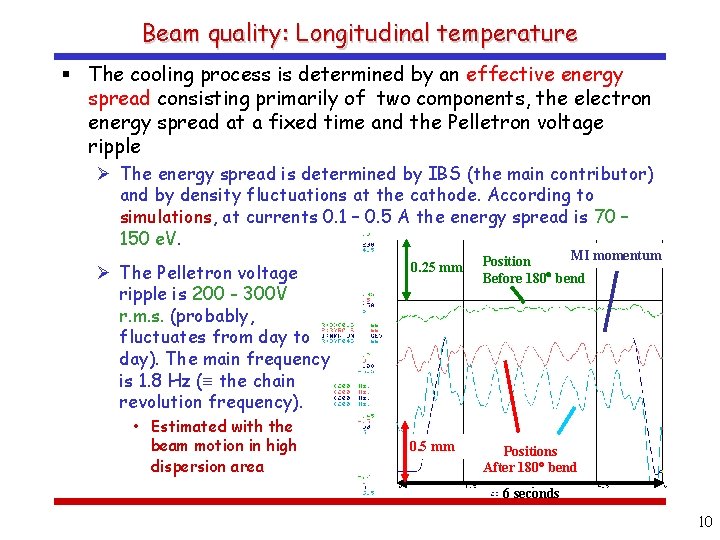
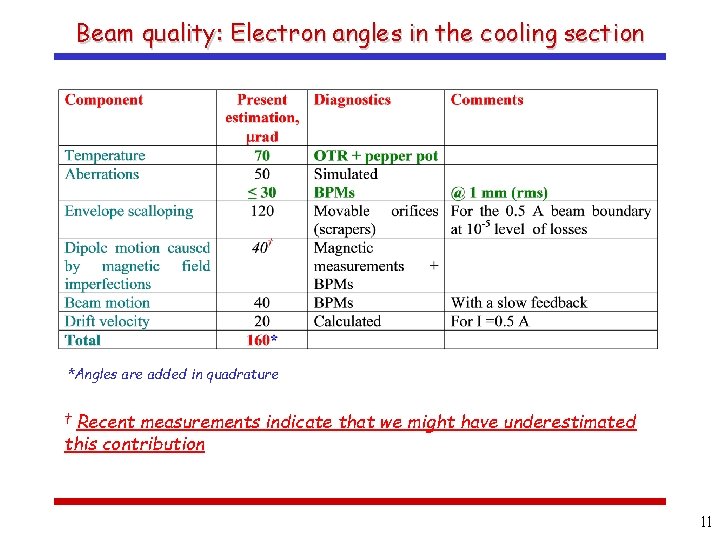
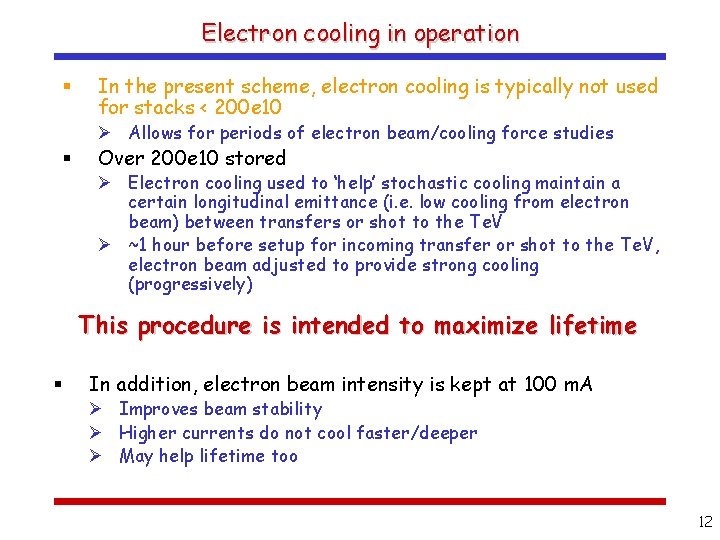
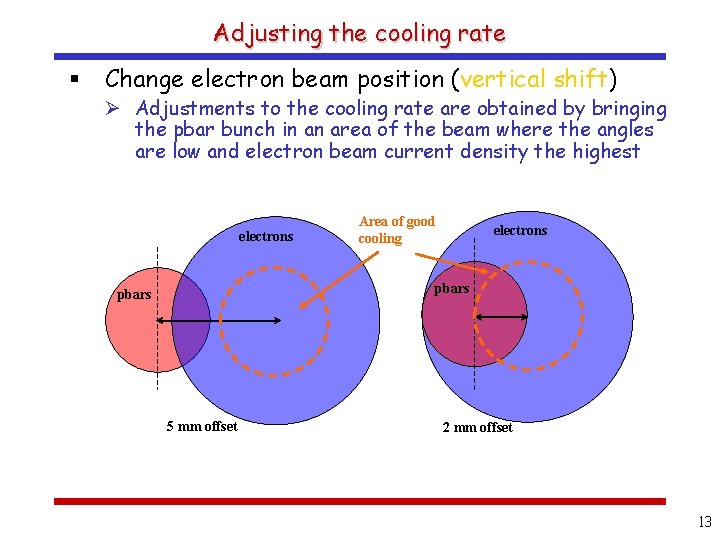
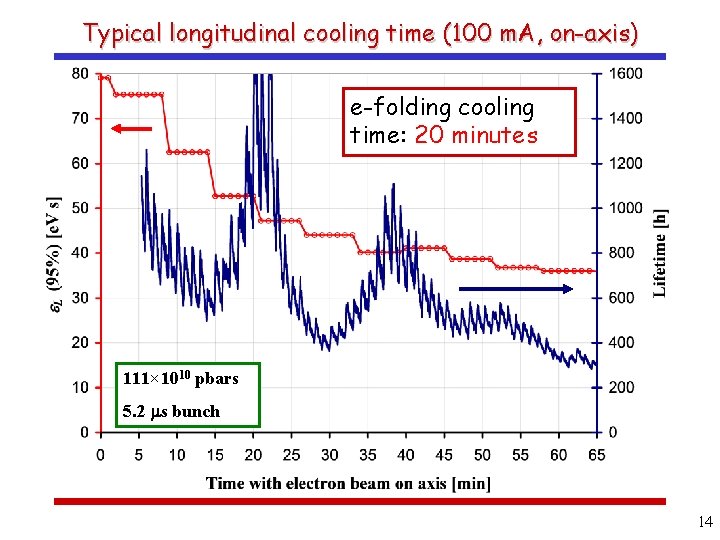
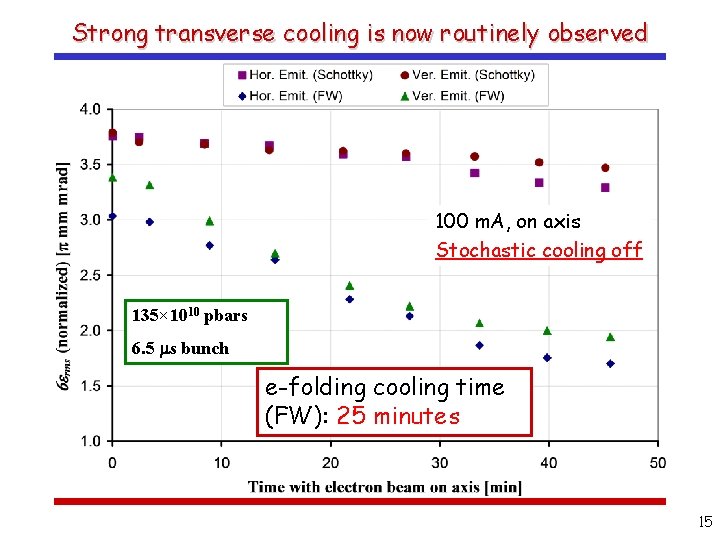
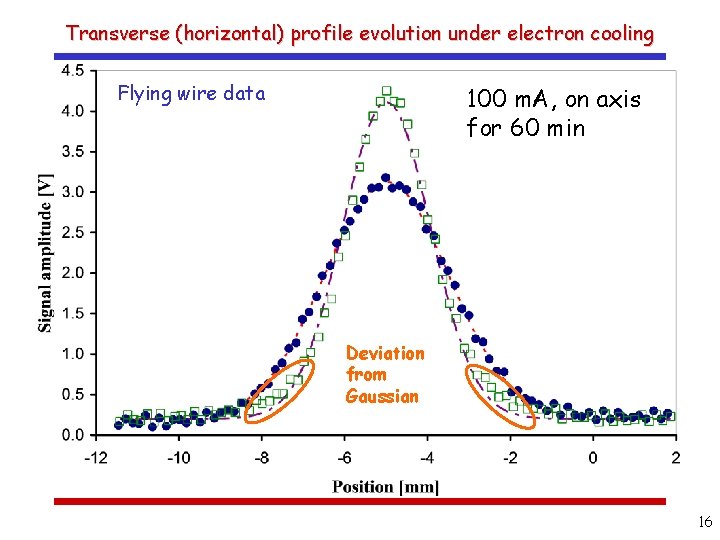
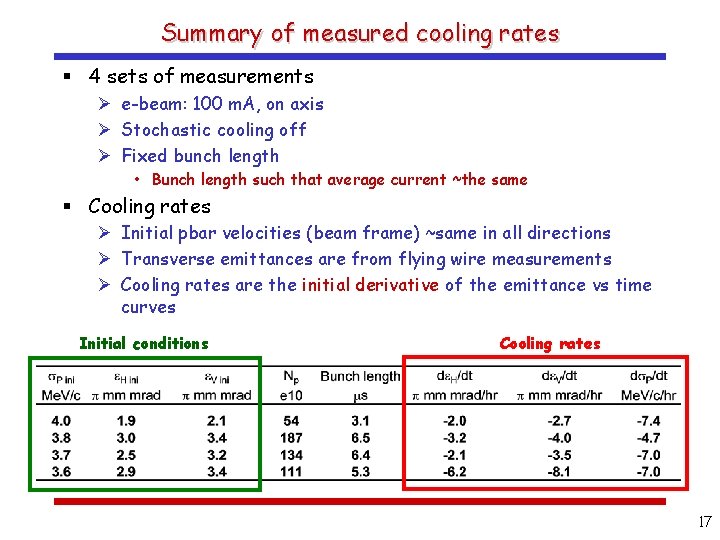
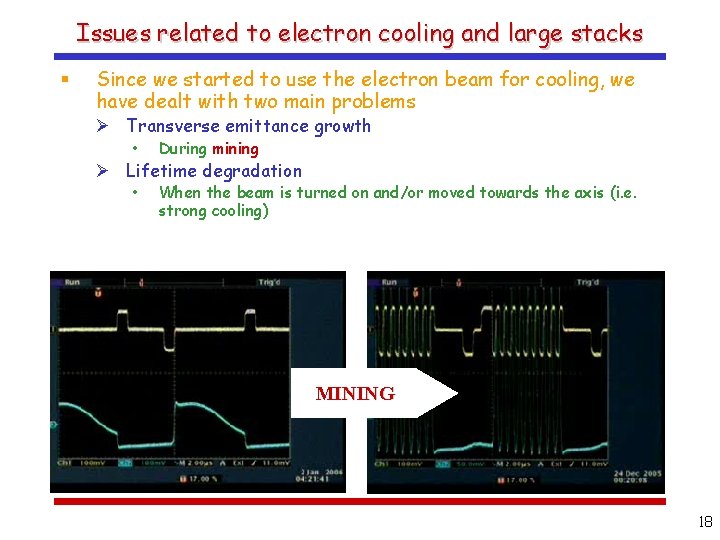
![Emittance growth during mining [Nov ‘ 05 measurements] pbars intensity for all measurements = Emittance growth during mining [Nov ‘ 05 measurements] pbars intensity for all measurements =](https://slidetodoc.com/presentation_image_h2/9b8bce8d2617e696f7981d5c4007db4e/image-19.jpg)
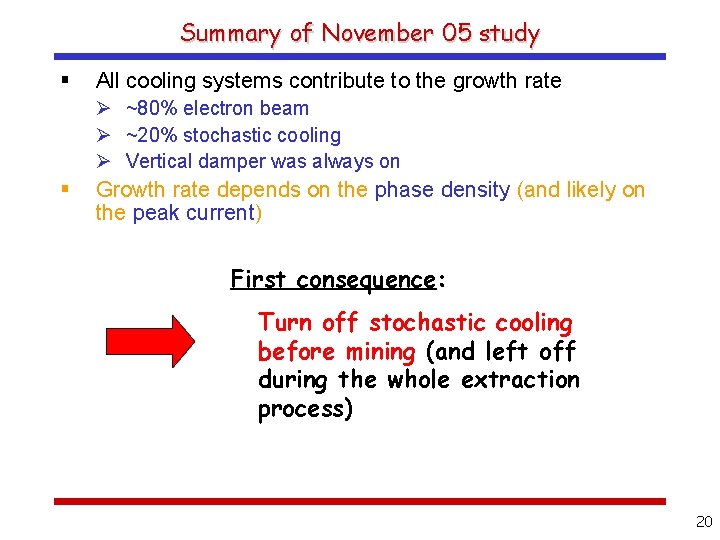
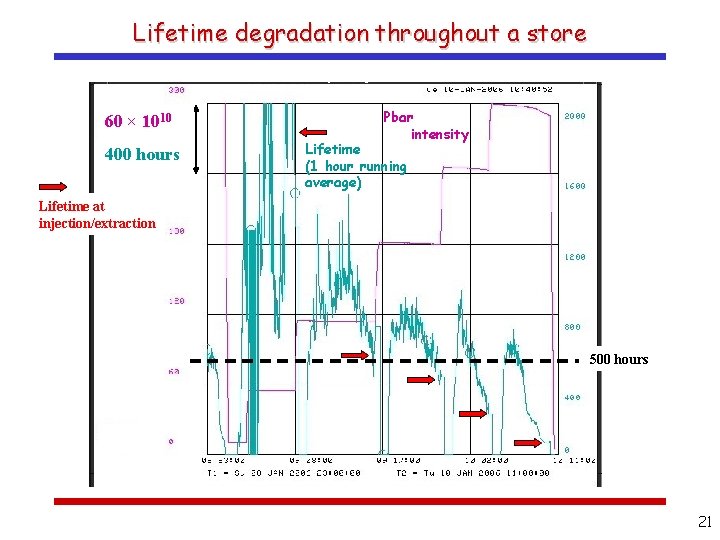
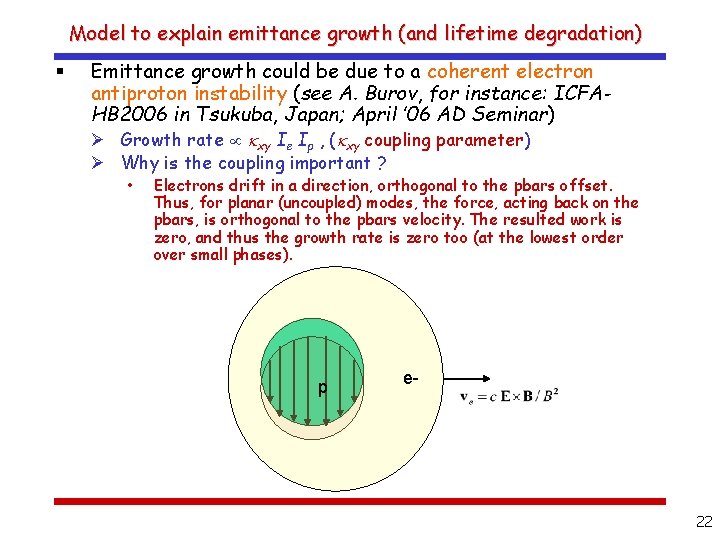
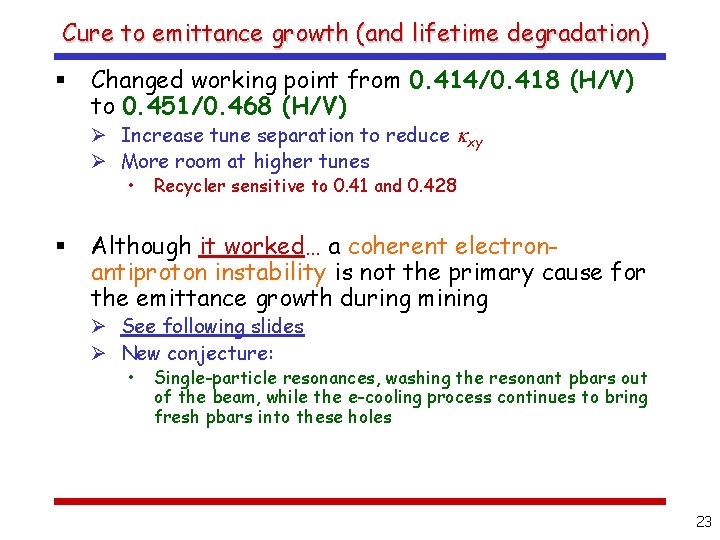
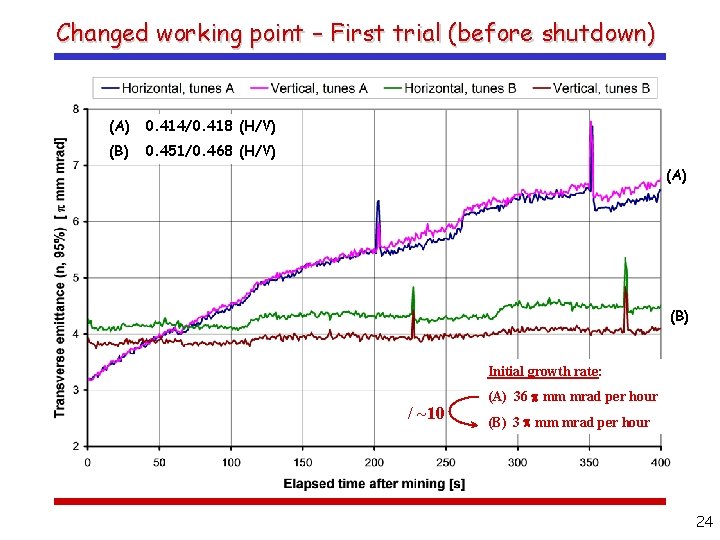
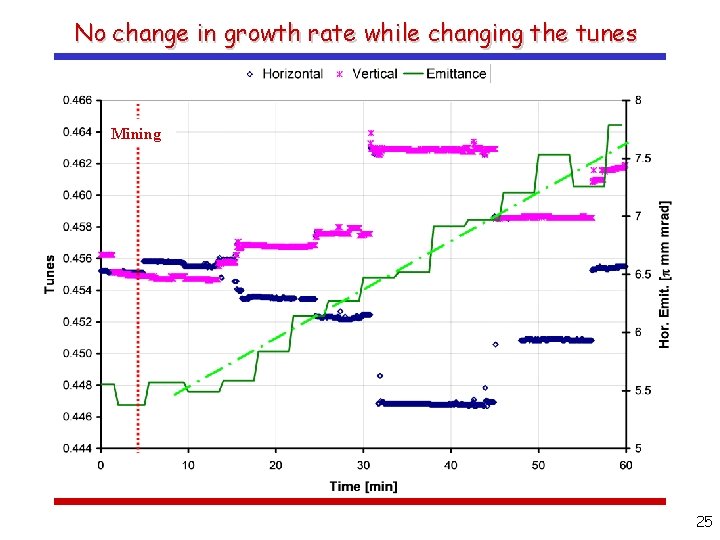
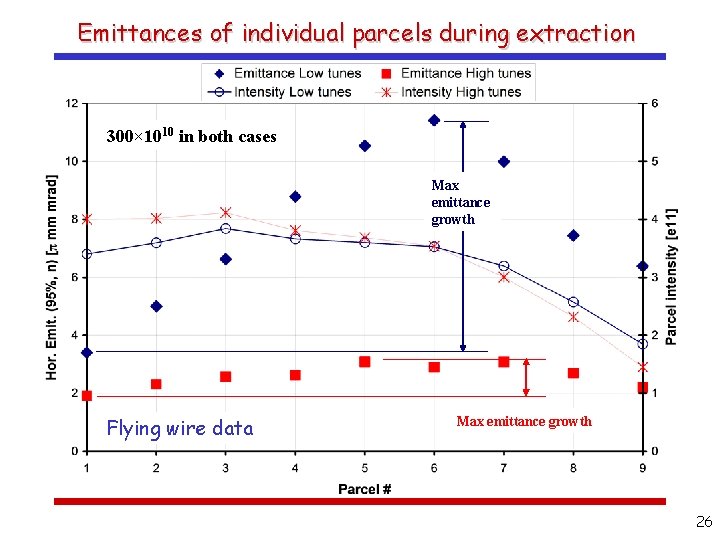
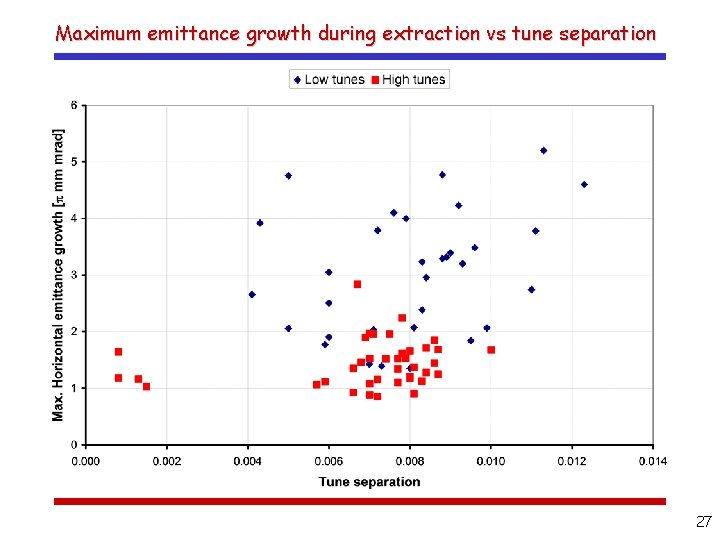
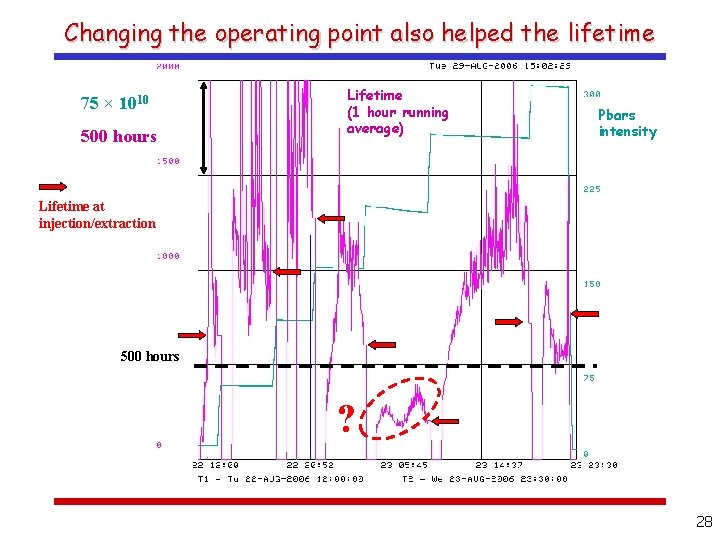
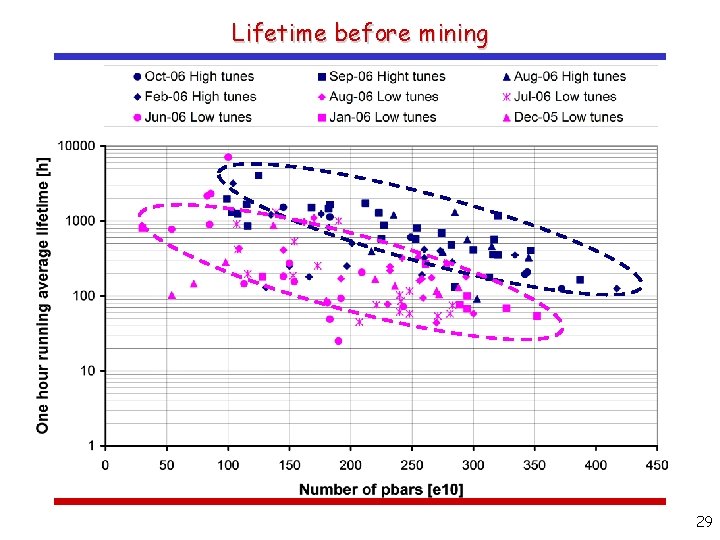
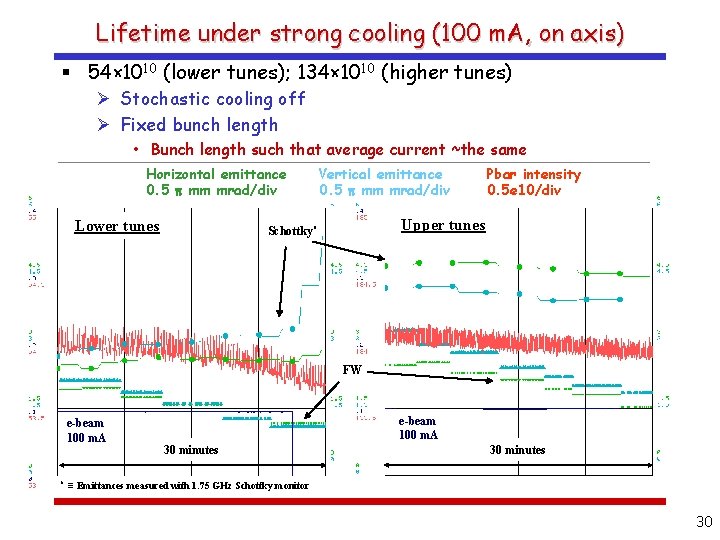
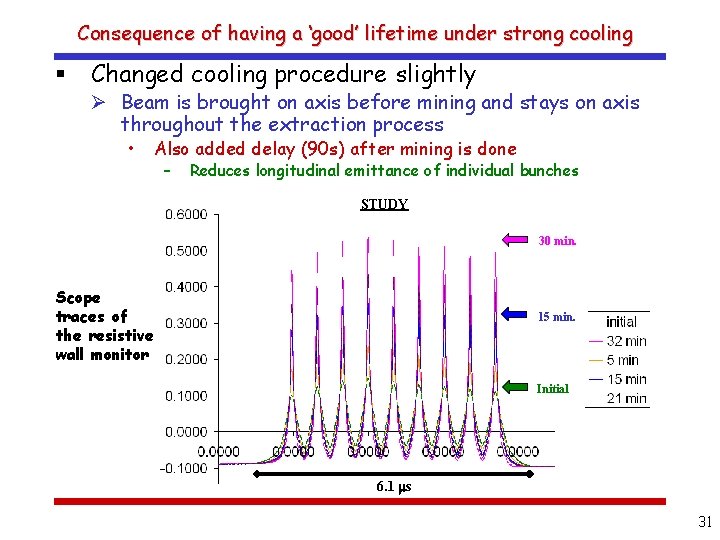
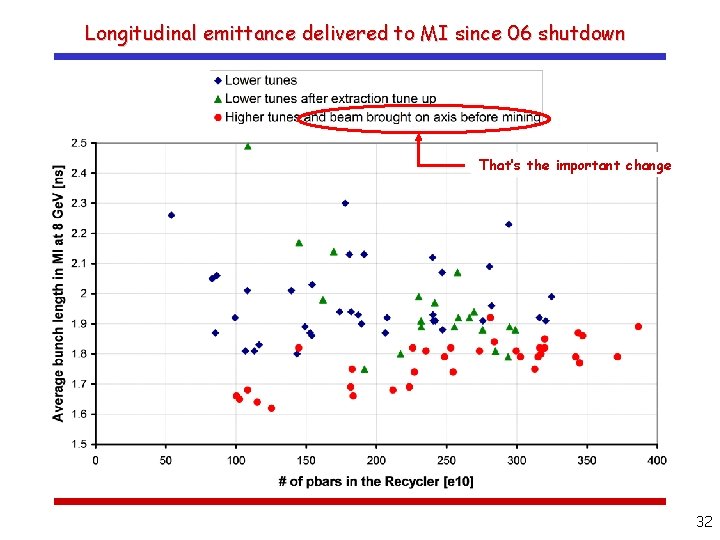
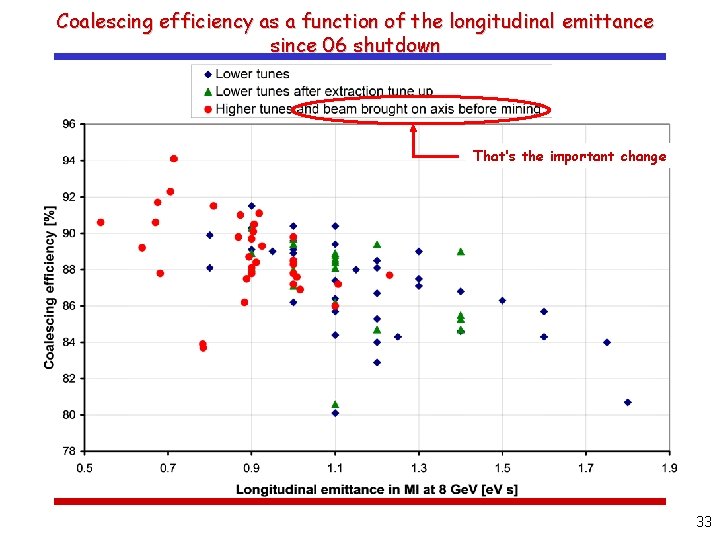
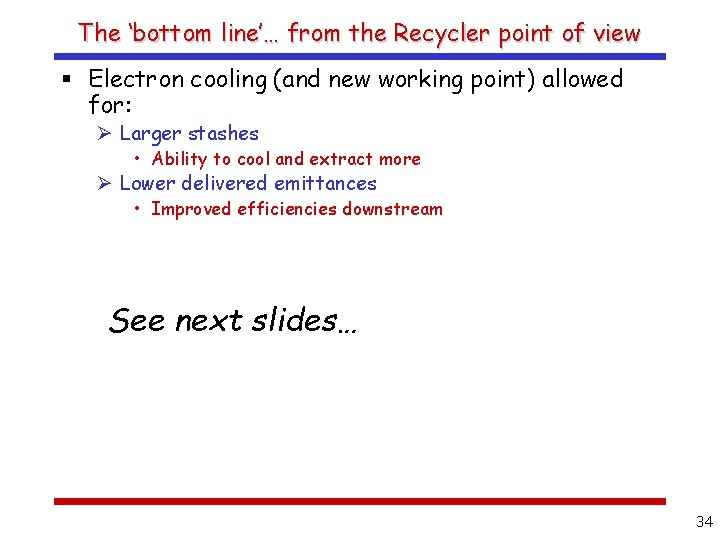
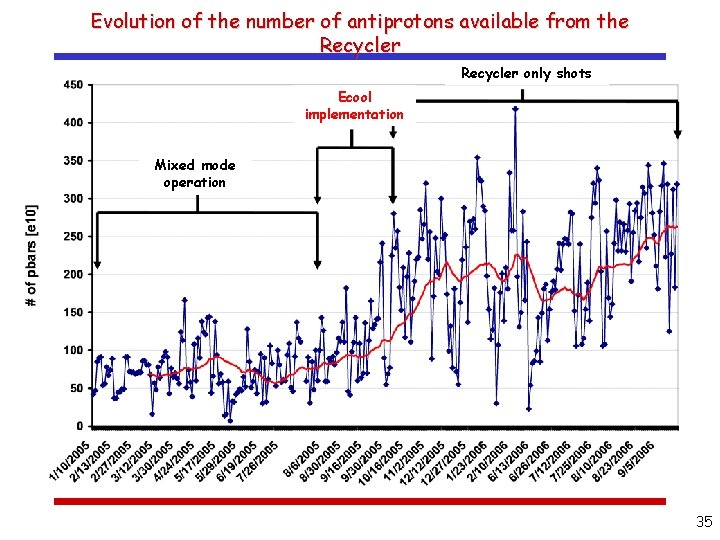
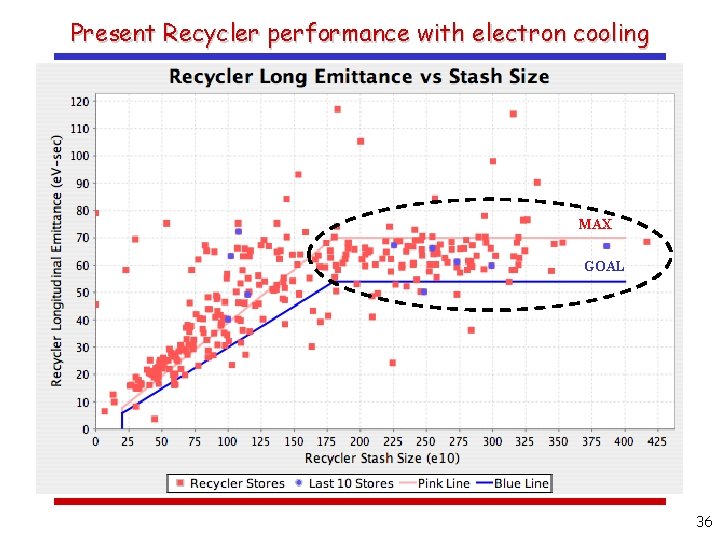
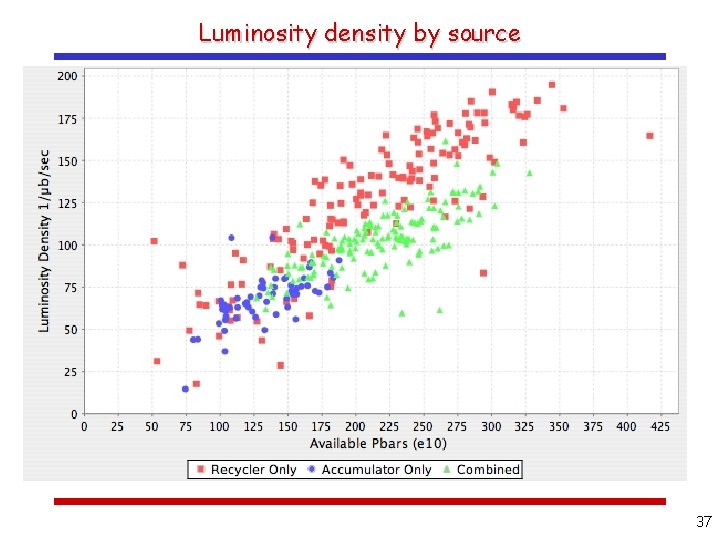
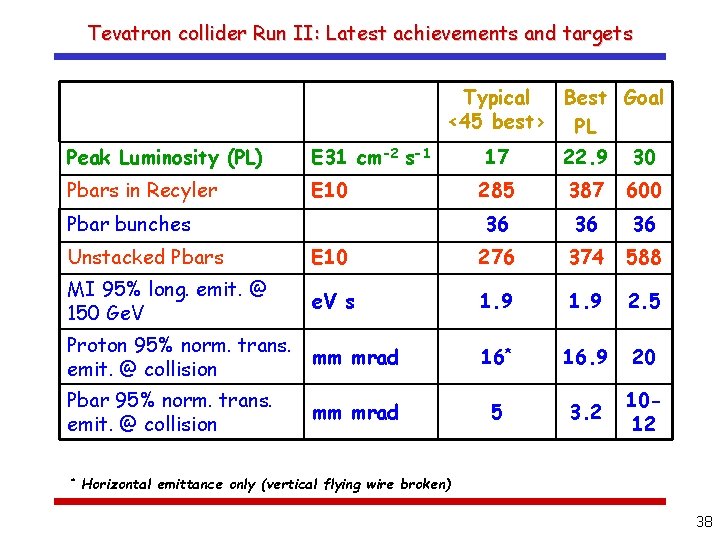
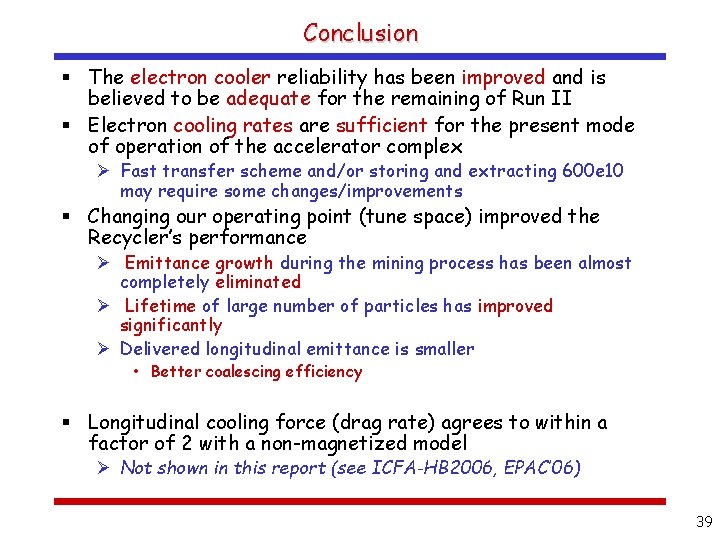
- Slides: 39
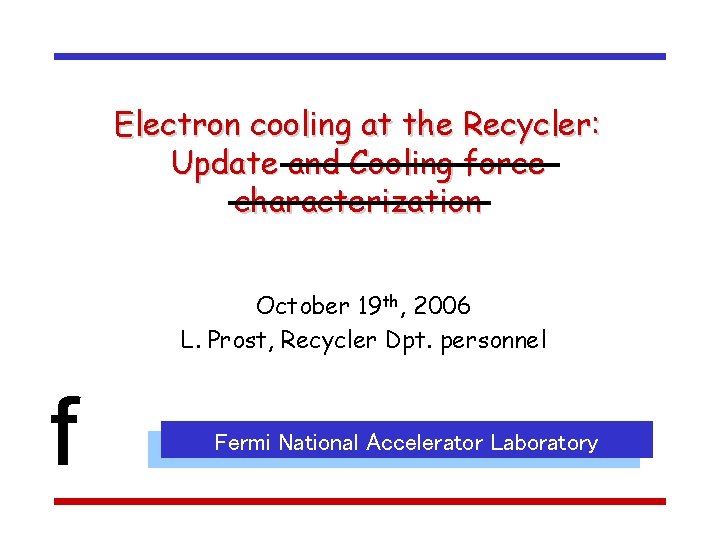
Electron cooling at the Recycler: Update and Cooling force characterization October 19 th, 2006 L. Prost, Recycler Dpt. personnel Fermi National Accelerator Laboratory
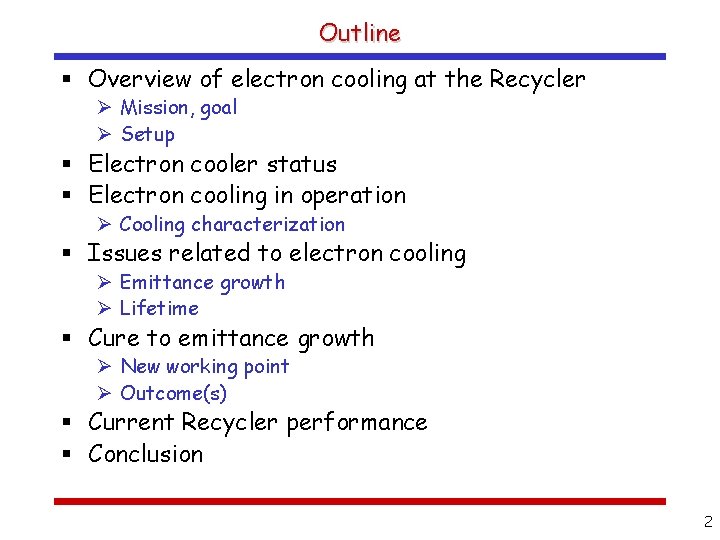
Outline § Overview of electron cooling at the Recycler Ø Mission, goal Ø Setup § Electron cooler status § Electron cooling in operation Ø Cooling characterization § Issues related to electron cooling Ø Emittance growth Ø Lifetime § Cure to emittance growth Ø New working point Ø Outcome(s) § Current Recycler performance § Conclusion 2
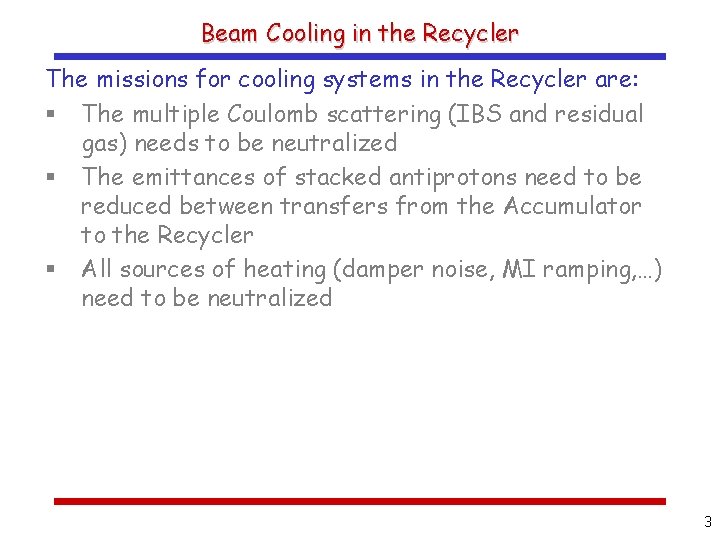
Beam Cooling in the Recycler The missions for cooling systems in the Recycler are: § The multiple Coulomb scattering (IBS and residual gas) needs to be neutralized § The emittances of stacked antiprotons need to be reduced between transfers from the Accumulator to the Recycler § All sources of heating (damper noise, MI ramping, …) need to be neutralized 3
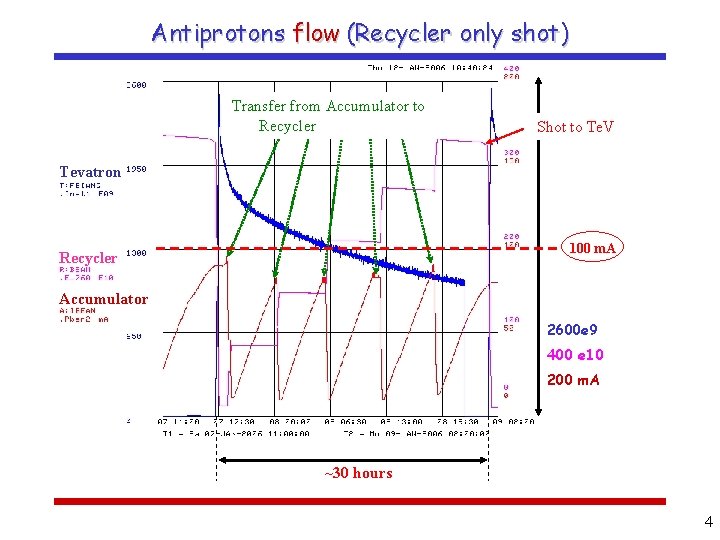
Antiprotons flow (Recycler only shot) Transfer from Accumulator to Recycler Shot to Te. V Tevatron 100 m. A Recycler Accumulator 2600 e 9 400 e 10 200 m. A ~30 hours 4
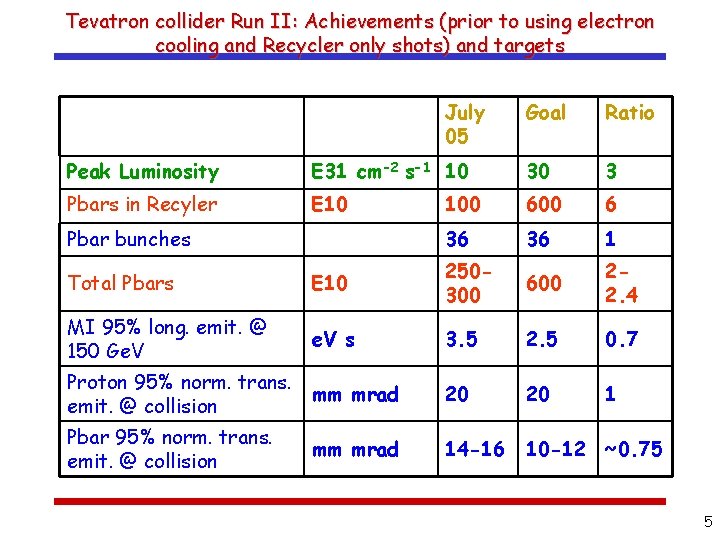
Tevatron collider Run II: Achievements (prior to using electron cooling and Recycler only shots) and targets July 05 Goal Ratio Peak Luminosity E 31 cm-2 s-1 10 30 3 Pbars in Recyler E 10 100 6 36 36 1 Pbar bunches Total Pbars E 10 250300 600 22. 4 MI 95% long. emit. @ 150 Ge. V s 3. 5 2. 5 0. 7 Proton 95% norm. trans. mm mrad emit. @ collision 20 20 1 Pbar 95% norm. trans. emit. @ collision 14 -16 10 -12 ~0. 75 mm mrad 5
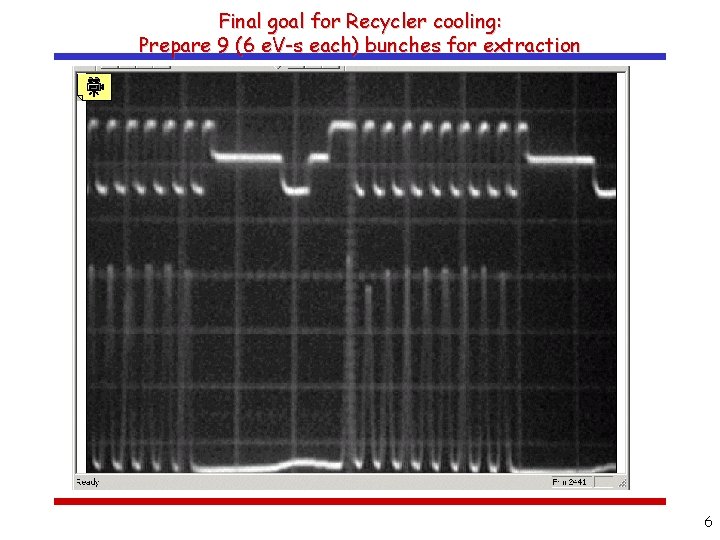
Final goal for Recycler cooling: Prepare 9 (6 e. V-s each) bunches for extraction 6
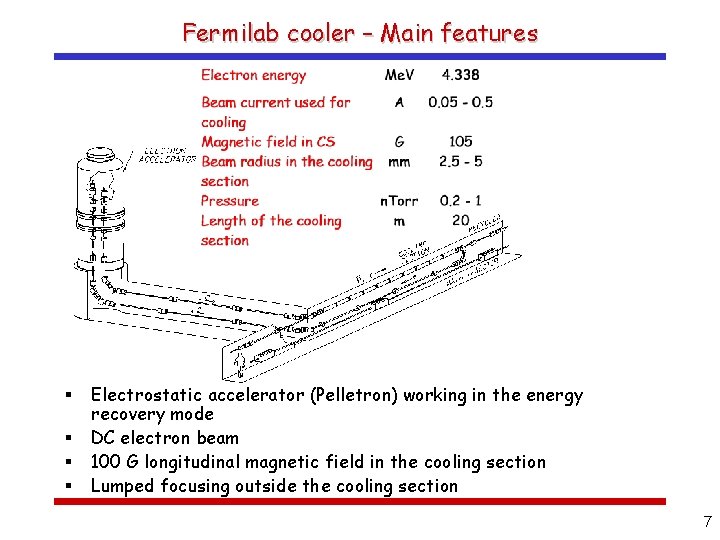
Fermilab cooler – Main features § § Electrostatic accelerator (Pelletron) working in the energy recovery mode DC electron beam 100 G longitudinal magnetic field in the cooling section Lumped focusing outside the cooling section 7
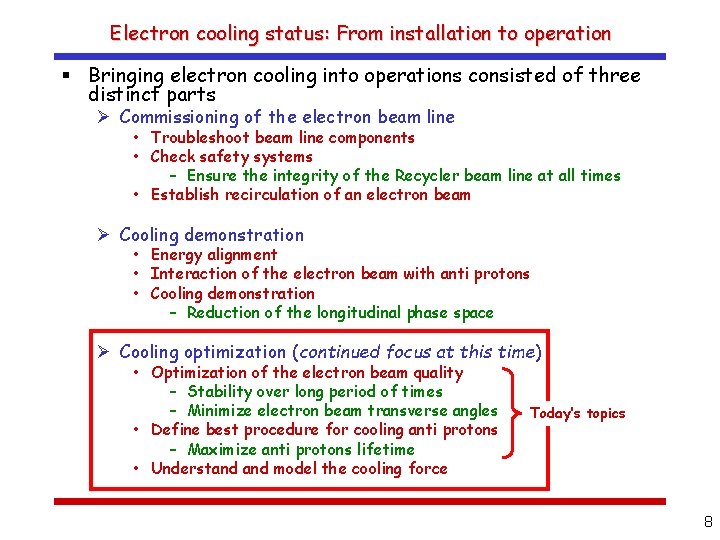
Electron cooling status: From installation to operation § Bringing electron cooling into operations consisted of three distinct parts Ø Commissioning of the electron beam line • Troubleshoot beam line components • Check safety systems – Ensure the integrity of the Recycler beam line at all times • Establish recirculation of an electron beam Ø Cooling demonstration • Energy alignment • Interaction of the electron beam with anti protons • Cooling demonstration – Reduction of the longitudinal phase space Ø Cooling optimization (continued focus at this time) • Optimization of the electron beam quality – Stability over long period of times – Minimize electron beam transverse angles • Define best procedure for cooling anti protons – Maximize anti protons lifetime • Understand model the cooling force Today’s topics 8
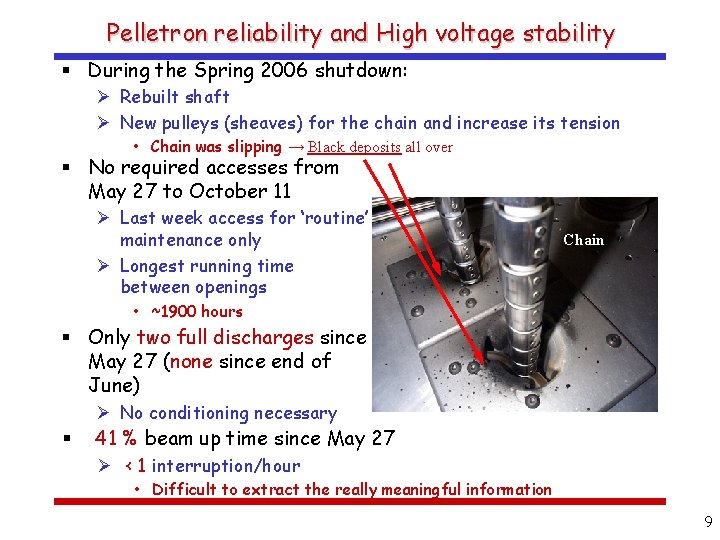
Pelletron reliability and High voltage stability § During the Spring 2006 shutdown: Ø Rebuilt shaft Ø New pulleys (sheaves) for the chain and increase its tension • Chain was slipping → Black deposits all over § No required accesses from May 27 to October 11 Ø Last week access for ‘routine’ maintenance only Ø Longest running time between openings Chain • ~1900 hours § Only two full discharges since May 27 (none since end of June) Ø No conditioning necessary § 41 % beam up time since May 27 Ø < 1 interruption/hour • Difficult to extract the really meaningful information 9
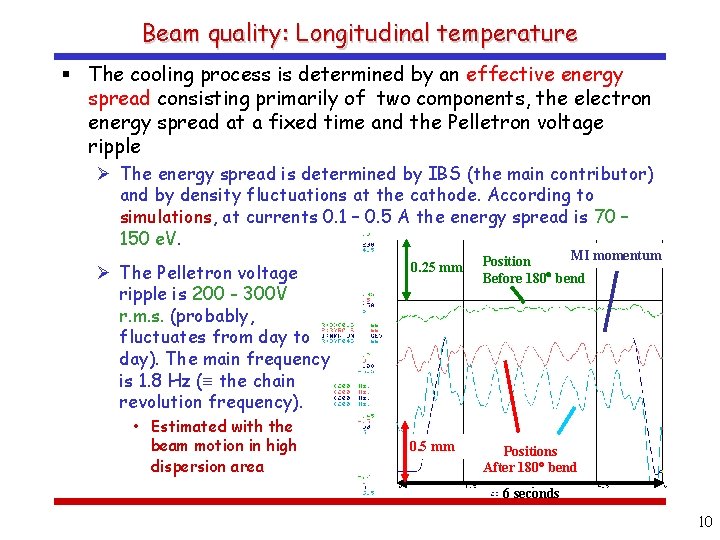
Beam quality: Longitudinal temperature § The cooling process is determined by an effective energy spread consisting primarily of two components, the electron energy spread at a fixed time and the Pelletron voltage ripple Ø The energy spread is determined by IBS (the main contributor) and by density fluctuations at the cathode. According to simulations, at currents 0. 1 – 0. 5 A the energy spread is 70 – 150 e. V. Ø The Pelletron voltage ripple is 200 - 300 V r. m. s. (probably, fluctuates from day to day). The main frequency is 1. 8 Hz (≡ the chain revolution frequency). • Estimated with the beam motion in high dispersion area 0. 25 mm 0. 5 mm MI momentum Position Before 180 bend Positions After 180 bend 6 seconds 10
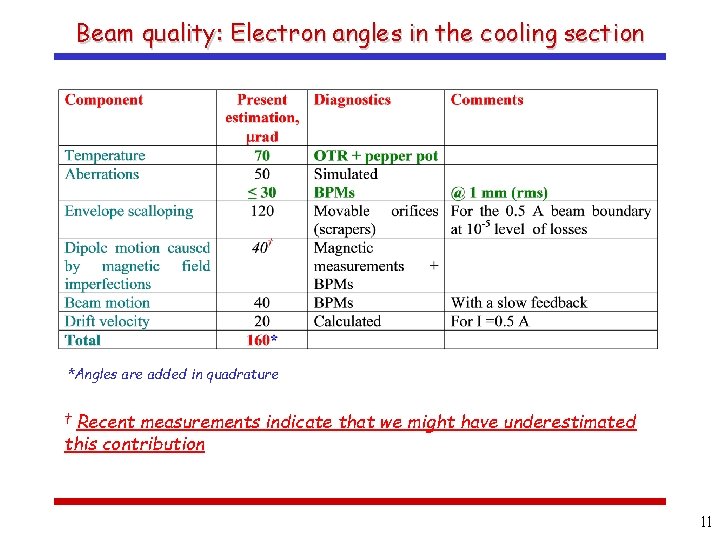
Beam quality: Electron angles in the cooling section *Angles are added in quadrature Recent measurements indicate that we might have underestimated this contribution † 11
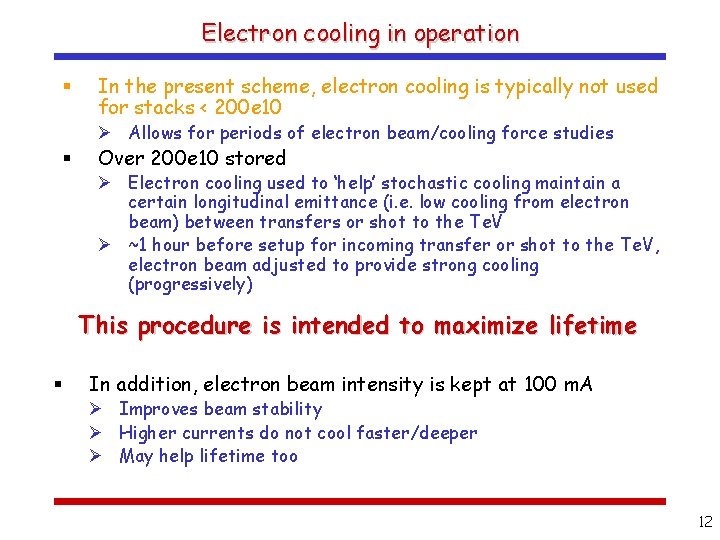
Electron cooling in operation § In the present scheme, electron cooling is typically not used for stacks < 200 e 10 Ø Allows for periods of electron beam/cooling force studies § Over 200 e 10 stored Ø Electron cooling used to ‘help’ stochastic cooling maintain a certain longitudinal emittance (i. e. low cooling from electron beam) between transfers or shot to the Te. V Ø ~1 hour before setup for incoming transfer or shot to the Te. V, electron beam adjusted to provide strong cooling (progressively) This procedure is intended to maximize lifetime § In addition, electron beam intensity is kept at 100 m. A Ø Improves beam stability Ø Higher currents do not cool faster/deeper Ø May help lifetime too 12
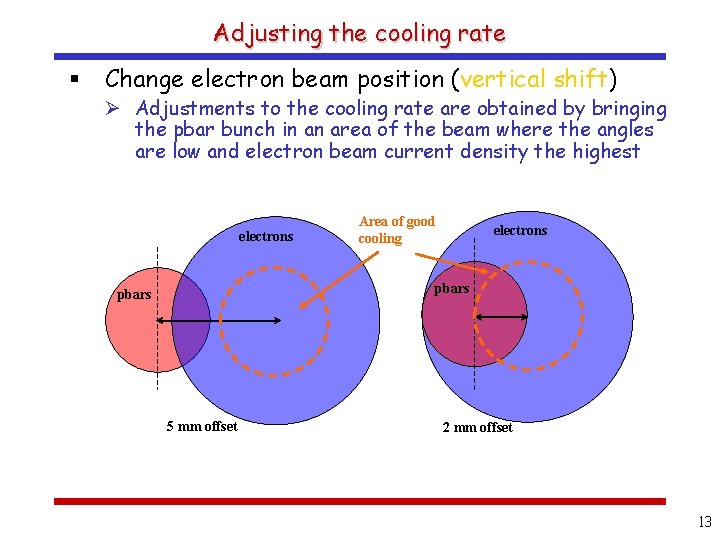
Adjusting the cooling rate § Change electron beam position (vertical shift) Ø Adjustments to the cooling rate are obtained by bringing the pbar bunch in an area of the beam where the angles are low and electron beam current density the highest electrons Area of good cooling electrons pbars 5 mm offset 2 mm offset 13
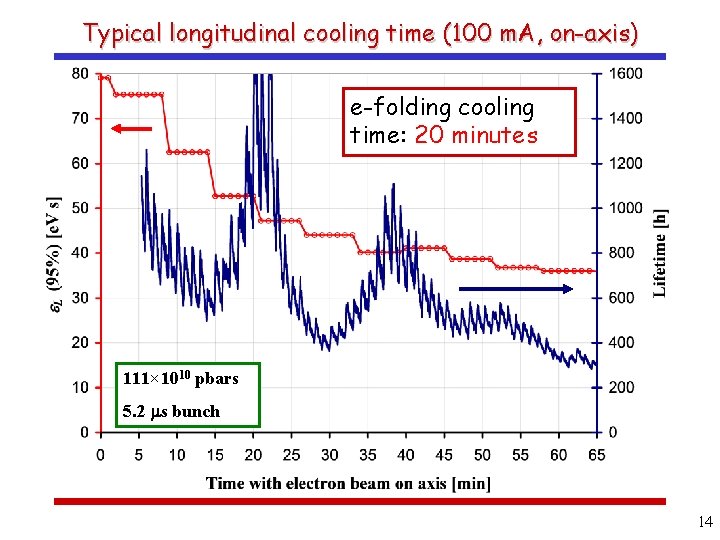
Typical longitudinal cooling time (100 m. A, on-axis) e-folding cooling time: 20 minutes 111× 1010 pbars 5. 2 ms bunch 14
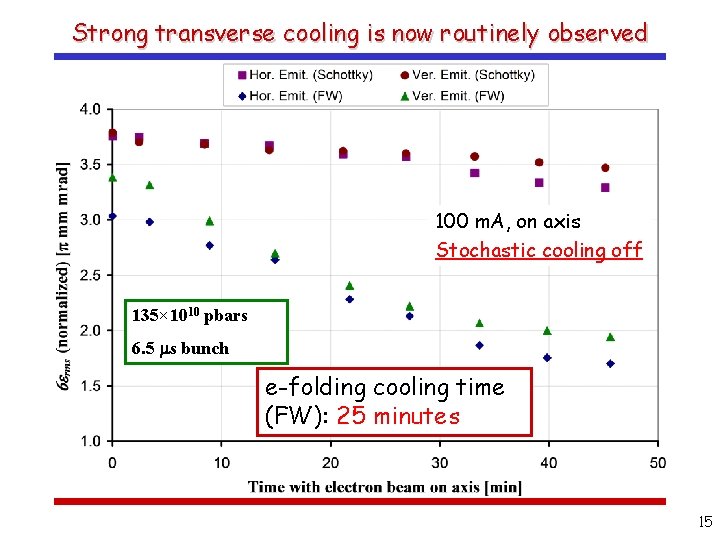
Strong transverse cooling is now routinely observed 100 m. A, on axis Stochastic cooling off 135× 1010 pbars 6. 5 ms bunch e-folding cooling time (FW): 25 minutes 15
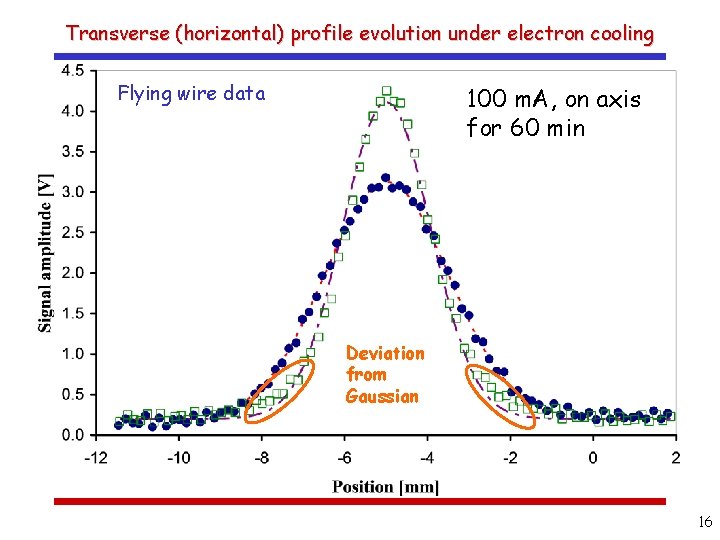
Transverse (horizontal) profile evolution under electron cooling Flying wire data 100 m. A, on axis for 60 min Deviation from Gaussian 16
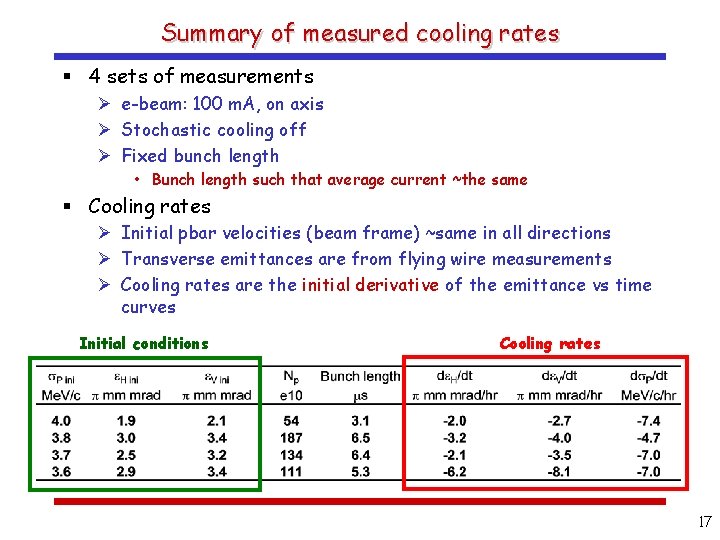
Summary of measured cooling rates § 4 sets of measurements Ø e-beam: 100 m. A, on axis Ø Stochastic cooling off Ø Fixed bunch length • Bunch length such that average current ~the same § Cooling rates Ø Initial pbar velocities (beam frame) ~same in all directions Ø Transverse emittances are from flying wire measurements Ø Cooling rates are the initial derivative of the emittance vs time curves Initial conditions Cooling rates 17
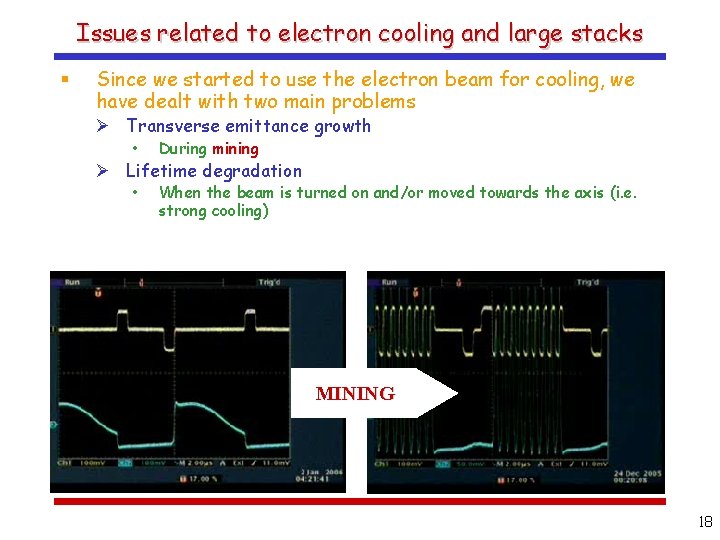
Issues related to electron cooling and large stacks § Since we started to use the electron beam for cooling, we have dealt with two main problems Ø Transverse emittance growth • During mining • When the beam is turned on and/or moved towards the axis (i. e. strong cooling) Ø Lifetime degradation MINING 18
![Emittance growth during mining Nov 05 measurements pbars intensity for all measurements Emittance growth during mining [Nov ‘ 05 measurements] pbars intensity for all measurements =](https://slidetodoc.com/presentation_image_h2/9b8bce8d2617e696f7981d5c4007db4e/image-19.jpg)
Emittance growth during mining [Nov ‘ 05 measurements] pbars intensity for all measurements = 97 e 10 19
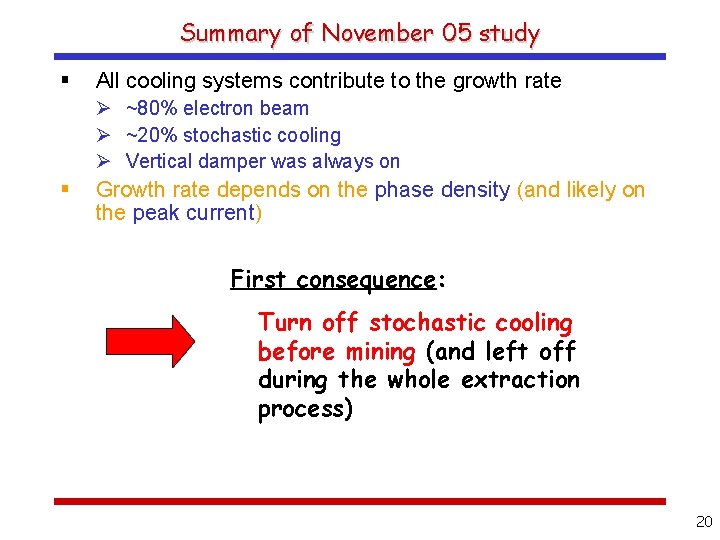
Summary of November 05 study § All cooling systems contribute to the growth rate Ø ~80% electron beam Ø ~20% stochastic cooling Ø Vertical damper was always on § Growth rate depends on the phase density (and likely on the peak current) First consequence: Turn off stochastic cooling before mining (and left off during the whole extraction process) 20
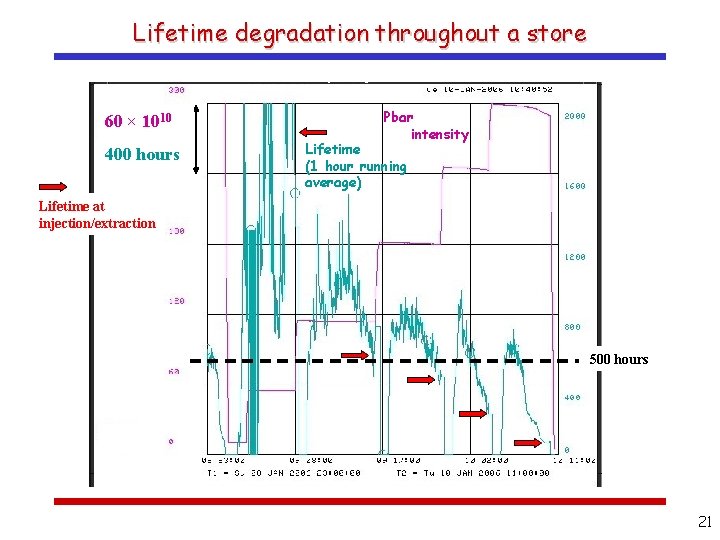
Lifetime degradation throughout a store 60 × 1010 400 hours Pbar intensity Lifetime (1 hour running average) Lifetime at injection/extraction 500 hours 21
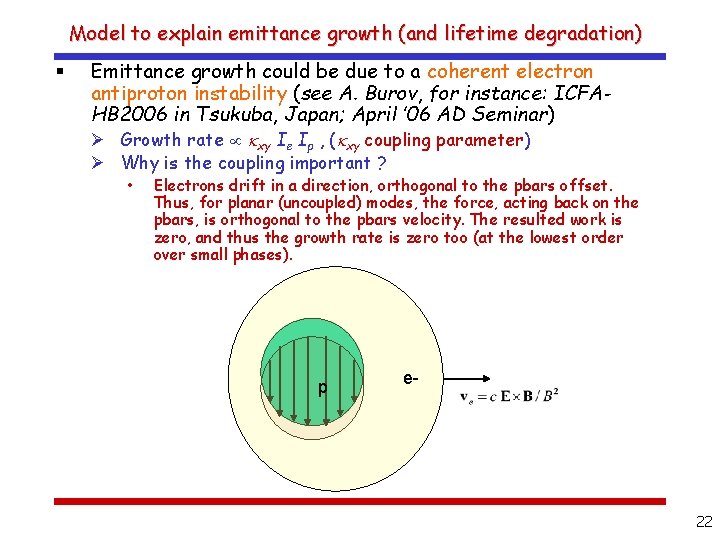
Model to explain emittance growth (and lifetime degradation) § Emittance growth could be due to a coherent electron antiproton instability (see A. Burov, for instance: ICFAHB 2006 in Tsukuba, Japan; April ’ 06 AD Seminar) Ø Growth rate kxy Ie Ip , (kxy coupling parameter) Ø Why is the coupling important ? • Electrons drift in a direction, orthogonal to the pbars offset. Thus, for planar (uncoupled) modes, the force, acting back on the pbars, is orthogonal to the pbars velocity. The resulted work is zero, and thus the growth rate is zero too (at the lowest order over small phases). p e- 22
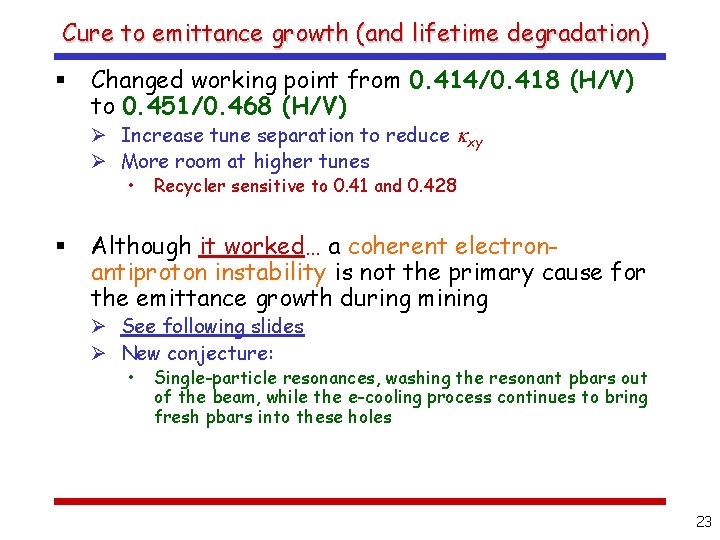
Cure to emittance growth (and lifetime degradation) § Changed working point from 0. 414/0. 418 (H/V) to 0. 451/0. 468 (H/V) Ø Increase tune separation to reduce kxy Ø More room at higher tunes • § Recycler sensitive to 0. 41 and 0. 428 Although it worked… a coherent electronantiproton instability is not the primary cause for the emittance growth during mining Ø See following slides Ø New conjecture: • Single-particle resonances, washing the resonant pbars out of the beam, while the e-cooling process continues to bring fresh pbars into these holes 23
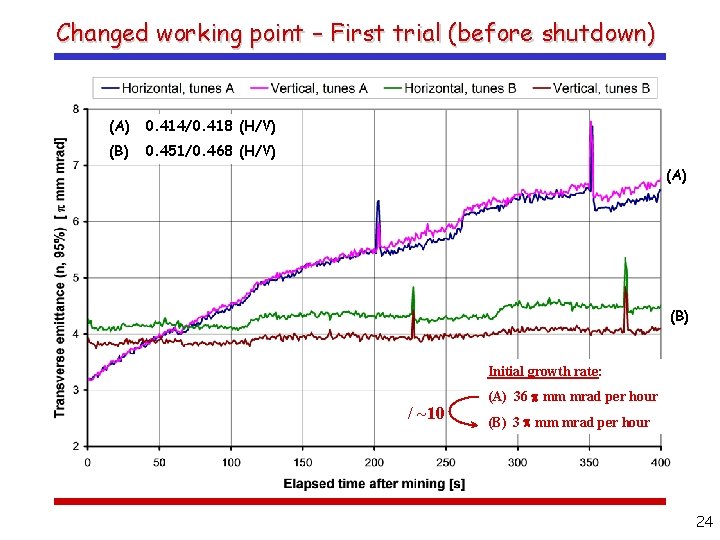
Changed working point – First trial (before shutdown) (A) 0. 414/0. 418 (H/V) (B) 0. 451/0. 468 (H/V) (A) (B) Initial growth rate: / ~10 (A) 36 p mm mrad per hour (B) 3 p mm mrad per hour 24
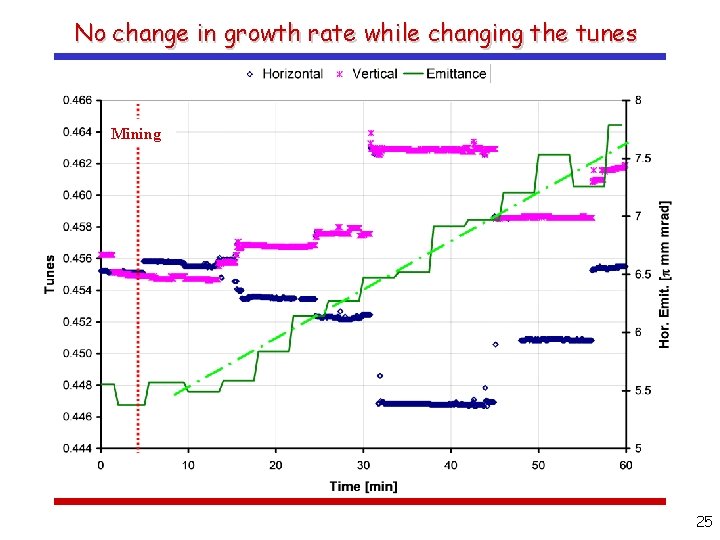
No change in growth rate while changing the tunes Mining 25
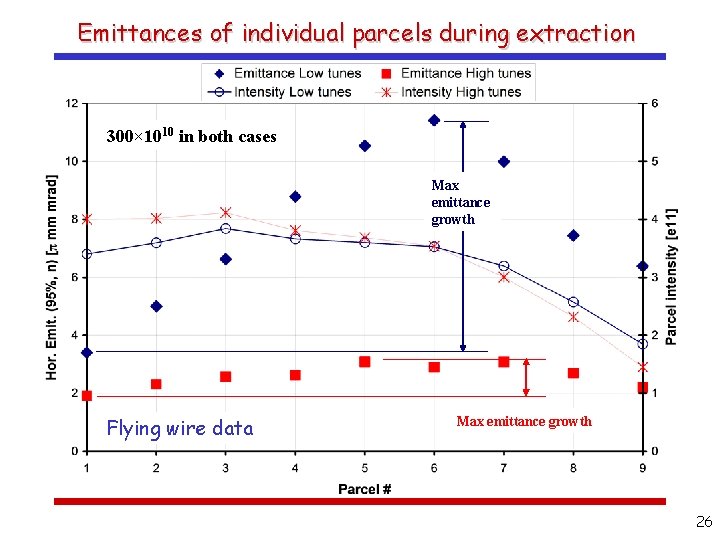
Emittances of individual parcels during extraction 300× 1010 in both cases Max emittance growth Flying wire data Max emittance growth 26
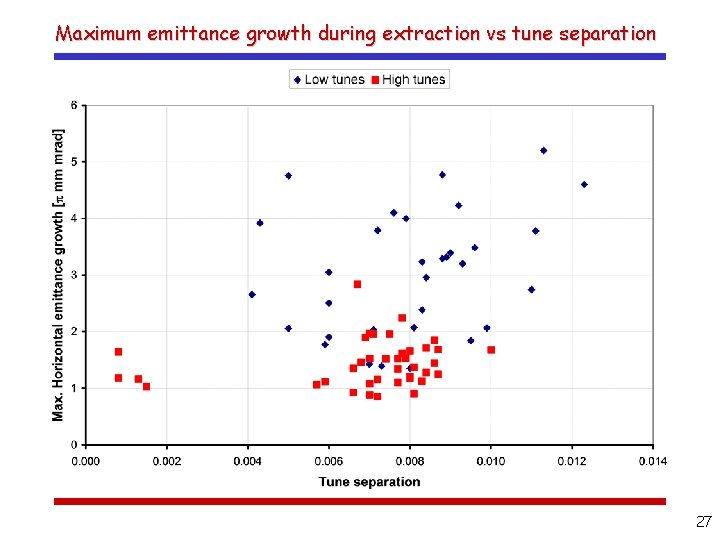
Maximum emittance growth during extraction vs tune separation 27
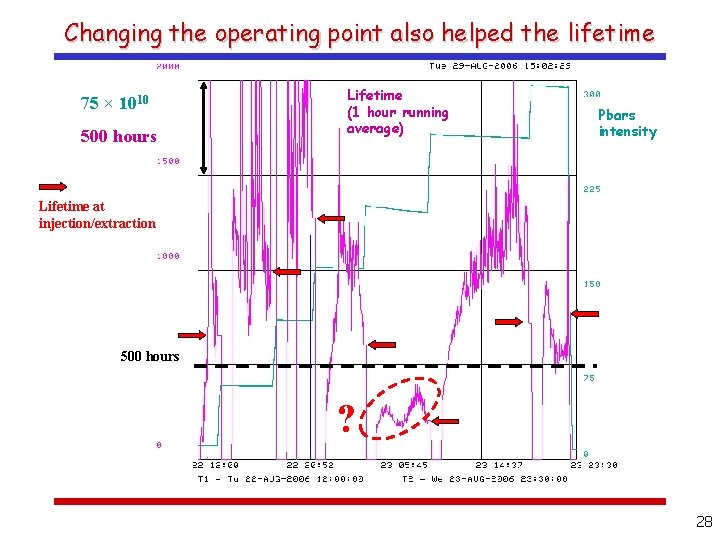
Changing the operating point also helped the lifetime 75 × 1010 500 hours Lifetime (1 hour running average) Pbars intensity Lifetime at injection/extraction 500 hours ? 28
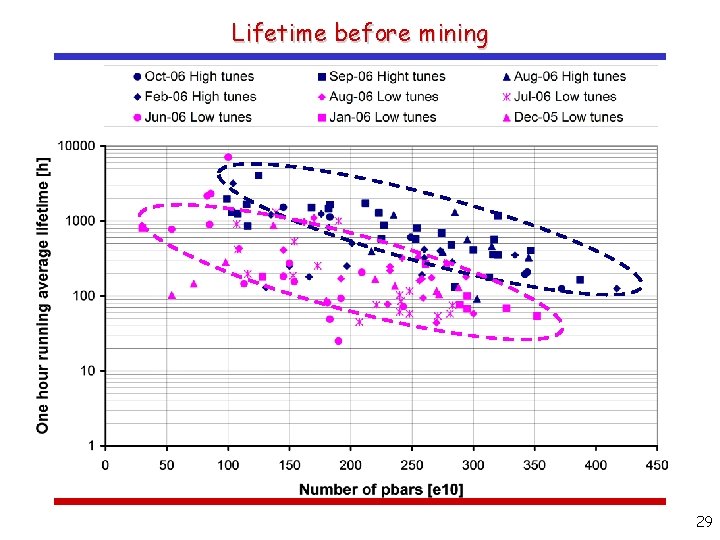
Lifetime before mining 29
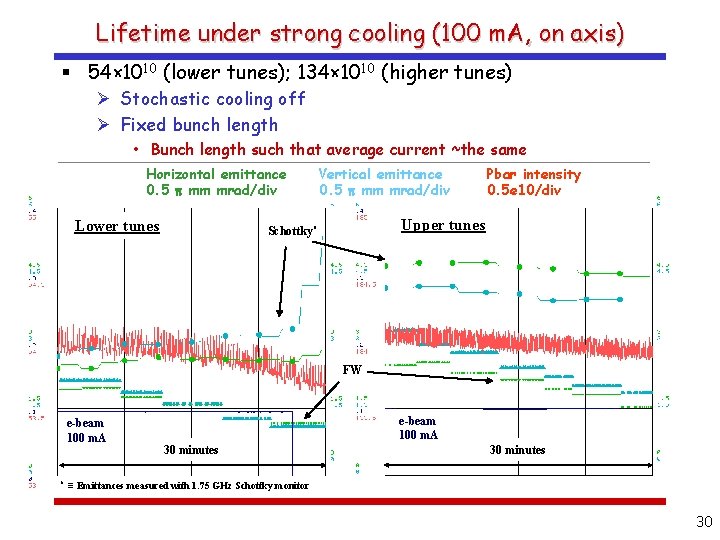
Lifetime under strong cooling (100 m. A, on axis) § 54× 1010 (lower tunes); 134× 1010 (higher tunes) Ø Stochastic cooling off Ø Fixed bunch length • Bunch length such that average current ~the same Horizontal emittance 0. 5 p mm mrad/div Lower tunes Vertical emittance 0. 5 p mm mrad/div Pbar intensity 0. 5 e 10/div Upper tunes Schottky* FW e-beam 100 m. A * e-beam 100 m. A 30 minutes ≡ Emittances measured with 1. 75 GHz Schottky monitor 30
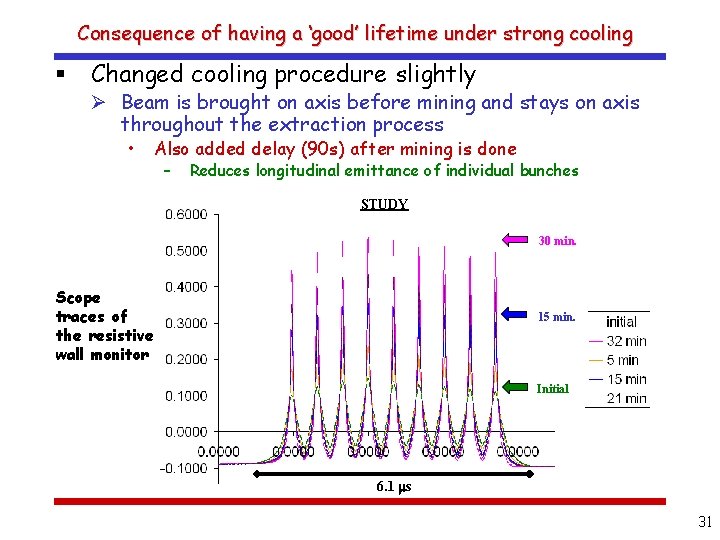
Consequence of having a ‘good’ lifetime under strong cooling § Changed cooling procedure slightly Ø Beam is brought on axis before mining and stays on axis throughout the extraction process • Also added delay (90 s) after mining is done – Reduces longitudinal emittance of individual bunches STUDY 30 min. Scope traces of the resistive wall monitor 15 min. Initial 6. 1 ms 31
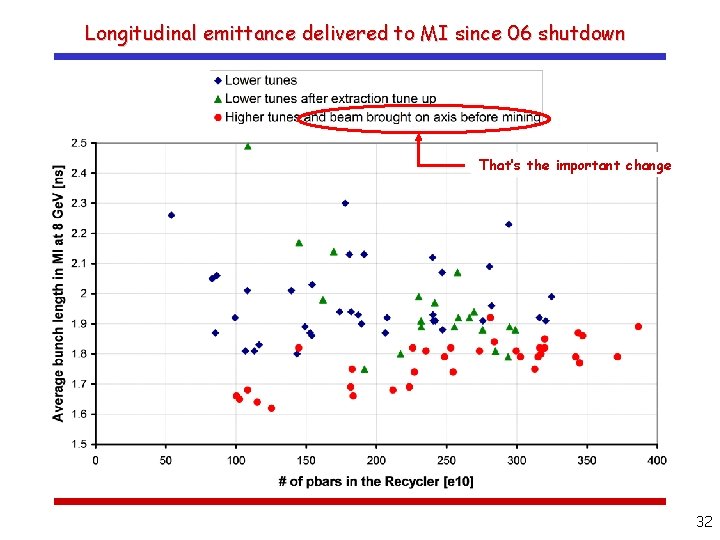
Longitudinal emittance delivered to MI since 06 shutdown That’s the important change 32
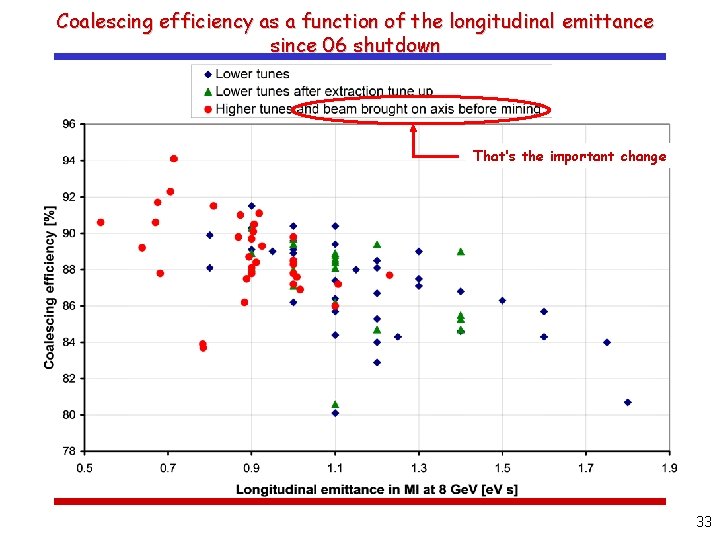
Coalescing efficiency as a function of the longitudinal emittance since 06 shutdown That’s the important change 33
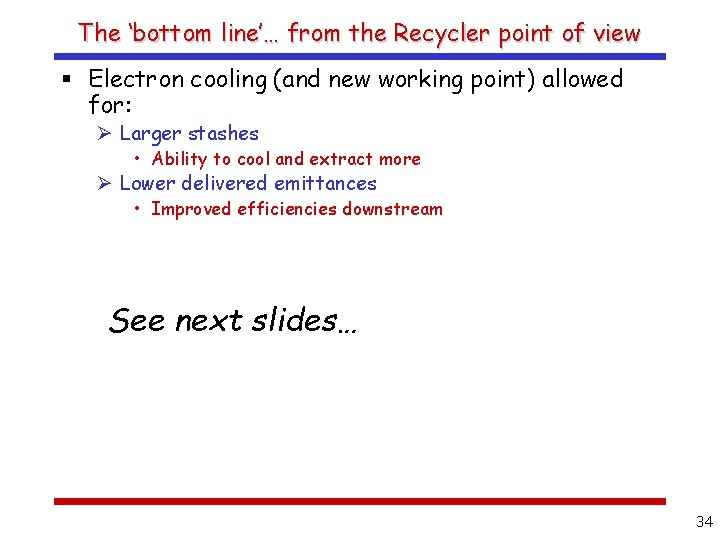
The ‘bottom line’… from the Recycler point of view § Electron cooling (and new working point) allowed for: Ø Larger stashes • Ability to cool and extract more Ø Lower delivered emittances • Improved efficiencies downstream See next slides… 34
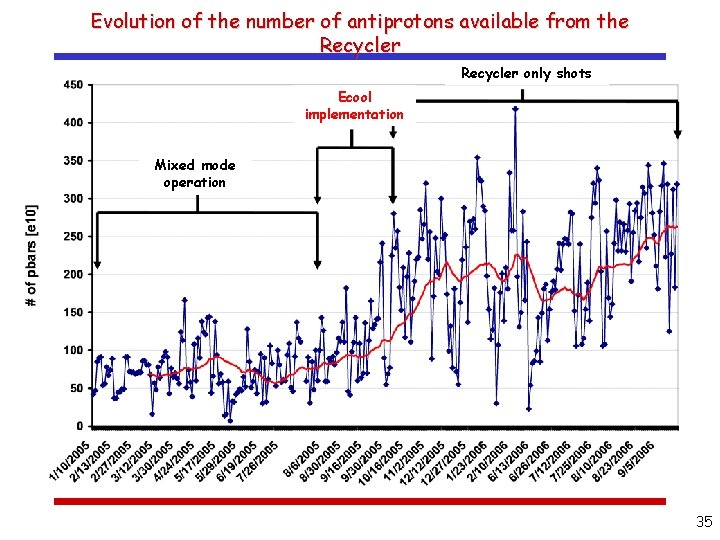
Evolution of the number of antiprotons available from the Recycler only shots Ecool implementation Mixed mode operation 35
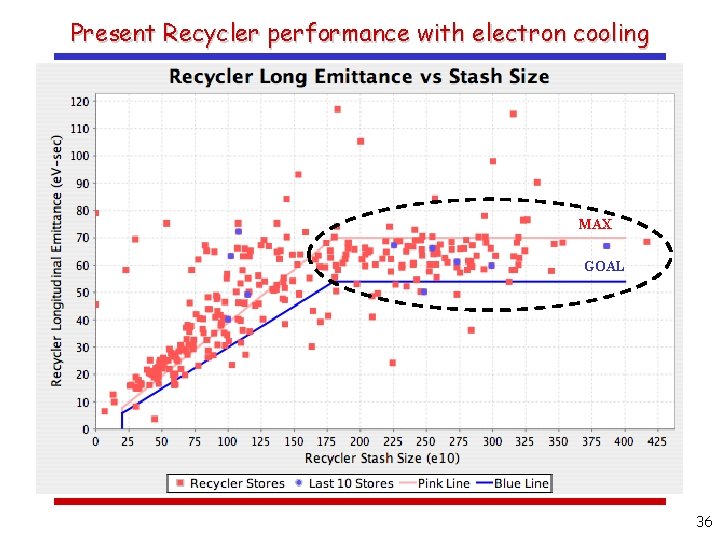
Present Recycler performance with electron cooling MAX GOAL 36
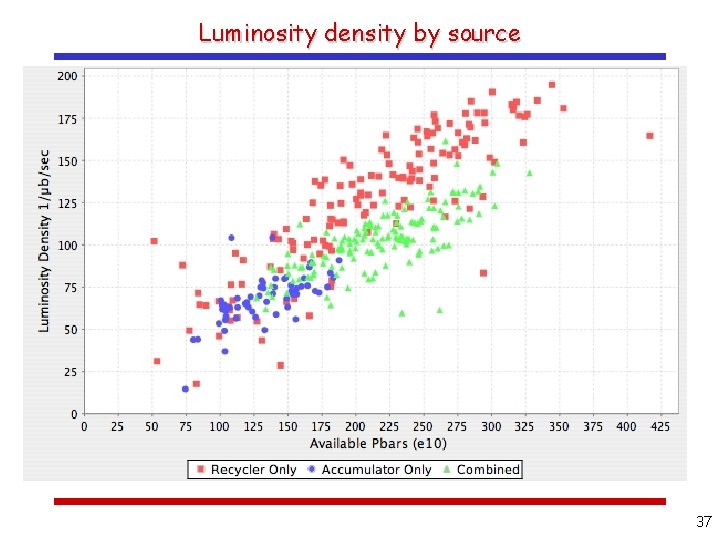
Luminosity density by source 37
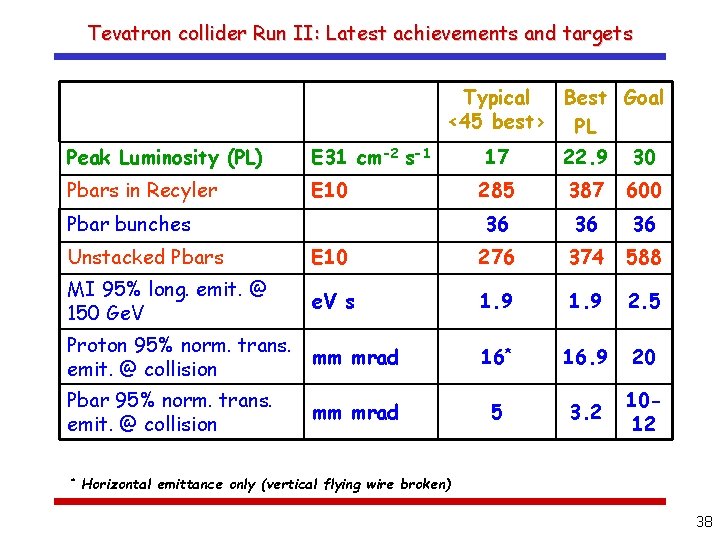
Tevatron collider Run II: Latest achievements and targets Typical Best Goal <45 best> PL Peak Luminosity (PL) E 31 cm-2 s-1 Pbars in Recyler E 10 Pbar bunches 17 22. 9 30 285 387 600 36 36 36 Unstacked Pbars E 10 276 374 588 MI 95% long. emit. @ 150 Ge. V s 1. 9 2. 5 16* 16. 9 20 5 3. 2 1012 Proton 95% norm. trans. mm mrad emit. @ collision Pbar 95% norm. trans. emit. @ collision * mm mrad Horizontal emittance only (vertical flying wire broken) 38
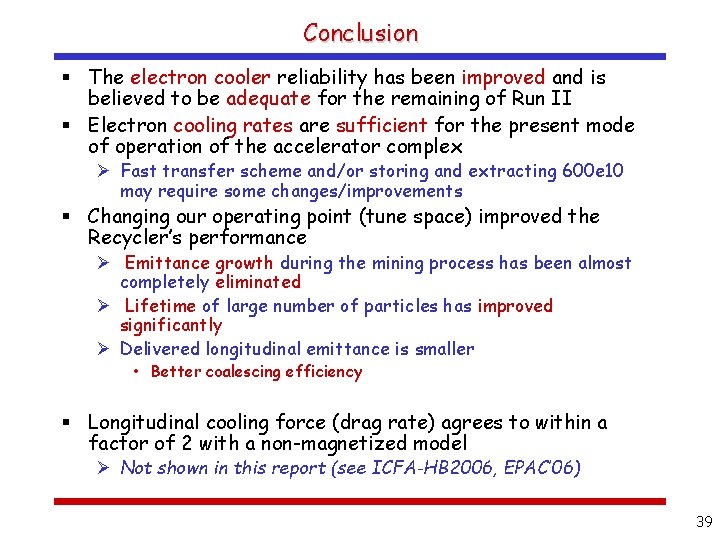
Conclusion § The electron cooler reliability has been improved and is believed to be adequate for the remaining of Run II § Electron cooling rates are sufficient for the present mode of operation of the accelerator complex Ø Fast transfer scheme and/or storing and extracting 600 e 10 may require some changes/improvements § Changing our operating point (tune space) improved the Recycler’s performance Ø Emittance growth during the mining process has been almost completely eliminated Ø Lifetime of large number of particles has improved significantly Ø Delivered longitudinal emittance is smaller • Better coalescing efficiency § Longitudinal cooling force (drag rate) agrees to within a factor of 2 with a non-magnetized model Ø Not shown in this report (see ICFA-HB 2006, EPAC’ 06) 39
What is an alternative of log based recovery
Ncr self checkout coin recycler error
Battery recycling process flowchart
Reputable isri scrap recycler
Cfm cash recycler
Recycler view
Develop and update food and beverage knowledge
Hát kết hợp bộ gõ cơ thể
Lp html
Bổ thể
Tỉ lệ cơ thể trẻ em
Chó sói
Chụp tư thế worms-breton
Alleluia hat len nguoi oi
Các môn thể thao bắt đầu bằng tiếng đua
Thế nào là hệ số cao nhất
Các châu lục và đại dương trên thế giới
Công thức tính độ biến thiên đông lượng
Trời xanh đây là của chúng ta thể thơ
Cách giải mật thư tọa độ
Phép trừ bù
Phản ứng thế ankan
Các châu lục và đại dương trên thế giới
Thể thơ truyền thống
Quá trình desamine hóa có thể tạo ra
Một số thể thơ truyền thống
Cái miệng xinh xinh thế chỉ nói điều hay thôi
Vẽ hình chiếu vuông góc của vật thể sau
Biện pháp chống mỏi cơ
đặc điểm cơ thể của người tối cổ
Ví dụ về giọng cùng tên
Vẽ hình chiếu đứng bằng cạnh của vật thể
Vẽ hình chiếu vuông góc của vật thể sau
Thẻ vin
đại từ thay thế
điện thế nghỉ
Tư thế ngồi viết
Diễn thế sinh thái là
Các loại đột biến cấu trúc nhiễm sắc thể
Bảng số nguyên tố